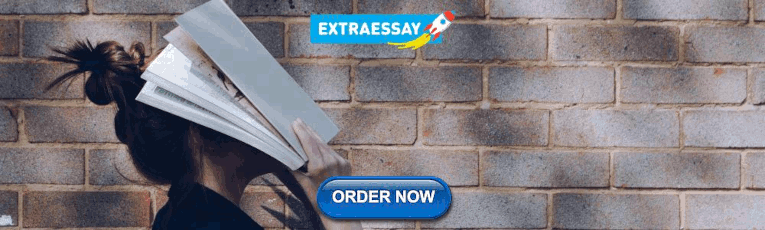
A study of the eutrophication of North Lake, Western Australia
Files and links (1).
Advertisement
Assessment of the eutrophication status of the Great Barrier Reef lagoon (Australia)
- Published: 17 November 2010
- Volume 106 , pages 281–302, ( 2011 )
Cite this article
- J. E. Brodie 1 ,
- M. Devlin 1 ,
- D. Haynes 2 nAff3 &
- J. Waterhouse 1
3415 Accesses
114 Citations
26 Altmetric
Explore all metrics
Current scientific consensus is that inshore regions of the central and southern Great Barrier Reef, Australia, are at risk of impacts from increased nutrient (as well as sediment and pesticide) loads delivered to Reef waters. Increases in the discharge of water quality contaminants to the Reef are largely a consequence of the expansion of agricultural practices in northern Queensland catchments following European settlement in the 1850s. In particular, the presence of elevated chlorophyll a and nutrient concentrations in many parts of the inshore Great Barrier Reef together with intense and extensive phytoplankton blooms following the discharge of nutrient-rich river flood waters suggest that the central and southern inshore area of the Great Barrier Reef is likely to be significantly impacted by elevated nutrient loads. The biological consequences of this are not fully quantified, but are likely to include changes in reef condition including hard and soft coral biodiversity, macroalgal abundance, hard coral cover and coral recruitment, as well as change in seagrass distribution and tissue nutrient status. Contemporary government policy is centered around promotion and funding of better catchment management practices to minimize the loss of catchment nutrients (both applied and natural) and the maintenance of a Reef wide water quality and ecosystem monitoring program. The monitoring program is designed to assess trends in uptake of management practice improvements and their associated impacts on water quality and ecosystem status over the next 10 years. A draft set of quantitative criteria to assess the eutrophication status of Great Barrier Reef waters is outlined for further discussion and refinement.
This is a preview of subscription content, log in via an institution to check access.
Access this article
Price includes VAT (Russian Federation)
Instant access to the full article PDF.
Rent this article via DeepDyve
Institutional subscriptions
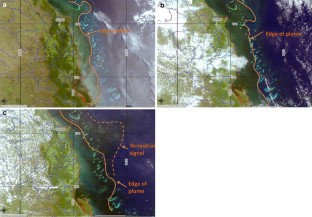
Similar content being viewed by others
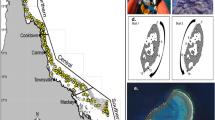
Changing dynamics of Great Barrier Reef hard coral cover in the Anthropocene
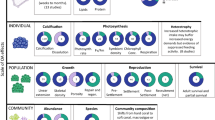
The indirect effects of ocean acidification on corals and coral communities
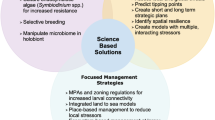
The coral conservation crisis: interacting local and global stressors reduce reef resiliency and create challenges for conservation solutions
Armour JD, Hateley LR, Pitt GL (2009) Catchment modelling of sediment, nitrogen and phosphorus nutrient loads with SedNet/ANNEX in the Tully-Murray basin. Mar Freshw Res 60:1091–1096
Article Google Scholar
Aronson RB, Precht WF (2000) Herbivory and algal dynamics on the coral reef at Discovery Bay, Jamaica. Limnol Oceanogr 45:251–255
Australian Government (2007) Labour’s reef rescue plan. Election 2007 Policy Document. http://www.alp.org.au/download/071028___labors_reef_rescue_plan.pdf
Babcock R, Davies P (1991) Effects of sedimentation on settlement of Acropora millepora . Coral Reefs 9:204–208
Bainbridge Z, Lewis S, Brodie J (2007) Sediment and nutrient exports for the Burdekin River catchment, NQ: a comparison of monitoring and modelling data. In: MODSIM 2007. International congress on modelling and simulation. Modelling and Simulation Society of Australia and New Zealand, 10–13th December 2007, pp 874–880
Bainbridge ZT, Brodie JE, Lewis SE, Waterhouse J, Wilkinson SN (2009) Utilising catchment modelling as a tool for monitoring Reef Rescue outcomes in the Great Barrier Reef catchment area. In: Amderssen B et al (eds) 18th IMACS world congress—MODSIM international congress on modelling and simulation, 13–17 July 2009, Cairns, Australia. ISBN: 978-0-9758400-7-8. http://mssanz.org.au/modsim09
Bell PRF (1991) The status of eutrophication in the Great Barrier Reef lagoon. Mar Pollut Bull 23:89–93
Bell PRF (1992) Eutrophication and coral reefs—some examples in the Great Barrier Reef lagoon. Water Res 28:553–568
Bell PRF, Elmetri I (1995) Ecological indicators of large-scale eutrophication in the Great Barrier Reef lagoon. Ambio 24:208–215
Google Scholar
Bell PRF, Elmetri I, Uwins P (1999) Nitrogen fixation by Trichodesmium spp. in the Central and Northern Great Barrier Reef Lagoon: relative importance of the fixed-nitrogen load. Mar Ecol Prog Ser 186:119–126
Bell PRF, Lapointe BR, Elmetri I (2007) Re-evaluation of ENCORE: support for the eutrophication threshold model for coral reefs. Ambio 36:416–424
Bellwood DR, Hughes TP, Folke C, Nystrom M (2004) Confronting the coral reef crises. Nature 429:827–833
Bellwood DR, Hoey AS, Ackerman JL, Depczynski M (2006) Coral bleaching, reef fish community phase shifts and the resilience of coral reefs. Glob Chang Biol 12(9):1587–1594
Bricker SB, Ferreira JG, Simas T (2003) An integrated methodology for assessment of estuarine trophic status. Ecol Modell 169:39–60
Brock RE, Smith SV (1983) Response of coral reef cryptofaunal communities to food and space. Coral Reefs 1:179–183
Brodie JE (1995) Nutrients and eutrophication in the Australian marine environment. In: Zann L, Sutton DC (eds) The State of the Marine Environment Report for Australia, technical annex 2, DEST report, Canberra, pp 1–29
Brodie JE (1997) Nutrients in the Great Barrier Reef region. In: Cosser P (ed) Nutrients in marine and estuarine environments. Australia: State of the Environment technical paper series (Estuaries and the Sea). Environment Australia, Canberra, pp 7–28
Brodie JE (2003) The Great Barrier Reef: 25 years of management as a large marine ecosystem. In: Hempel G, Sherman K (eds) Large marine ecosystems of the world: trends in exploration, protection, and research. pp 313–336
Brodie J, Bainbridge Z (2008) Water quality targets for the Burdekin WQIP. Australian Centre for Marine and Tropical Freshwater research report no. 08/05. James Cook University, Townsville
Brodie JE, Mitchell AW (2005) Nutrients in Australian tropical rivers: changes with agricultural development and implications for receiving environments. Mar Freshw Res 56(3):279–302
Brodie JE, Mitchell AW (2006) Sediments and nutrients in north Queensland tropical streams: changes with agricultural development and pristine condition status. CRC Reef Research Centre technical report no. 62. CRC Reef Research Centre, Townsville, 140 pp
Brodie JE, Waterhouse J (2009). Assessment of the relative risk of impacts of broad-scale agriculture on the Great Barrier Reef and priorities for investment under the Reef Protection Package. Stage 1 Report: April 2009. ACTFR technical report 09/17. Australian Centre for Tropical Freshwater Research, Townsville
Brodie J, Furnas M, Ghonim S, Haynes D, Mitchell A, Morris S, Waterhouse J, Yorkston H, Audas D, Lowe D, Ryan M (2001) Great Barrier Reef catchment water quality action plan. Great Barrier Reef Marine Park Authority, Townsville, 116 pp
Brodie J, Fabricius K, De’ath G, Okaji K (2005) Are increased nutrient inputs responsible for more outbreaks of crown-of-thorns starfish? An appraisal of the evidence. Mar Pollut Bull 51:266–278
Brodie J, De’ath G, Devlin M, Furnas M, Wright M (2007) Spatial and temporal patterns of near-surface chlorophyll a in the Great Barrier Reef lagoon. Mar Freshw Res 58:342–353
Brodie J, Binney J, Fabricius K, Gordon I, Hoegh-Guldberg O, Hunter H, O’Reagain P, Pearson R, Quirk M, Thorburn P, Waterhouse J, Webster I, Wilkinson S (2008a) Scientific consensus statement on water quality in the Great Barrier Reef. The State of Queensland (Department of Premier and Cabinet), Brisbane
Brodie J, Binney J, Fabricius K, Gordon I, Hoegh-Guldberg O, Hunter H, O’Reagain P, Pearson R, Quirk M, Thorburn P, Waterhouse J, Webster I, Wilkinson S (2008b) Synthesis of evidence to support the Scientific Consensus Statement on Water Quality in the Great Barrier Reef. The State of Queensland (Department of Premier and Cabinet), Brisbane
Brodie JE, Lewis SE, Bainbridge ZT, Mitchell A, Waterhouse J, Kroon F (2009a) Target setting for pollutant discharge management of rivers in the Great Barrier Reef catchment area. Mar Freshw Res 60:1141–1149
Brodie JE, Mitchell A, Waterhouse J (2009b) Regional assessment of the relative risk of the impacts of broad-scale agriculture on the Great Barrier Reef and priorities for investment under the Reef Protection Package. Stage 2 Report, July 2009. ACTFR technical report 09/30. Australian Centre for Tropical Freshwater Research, Townsville
Brodie J, Schroeder T, Rohde K, Faithful J, Masters B, Dekker A, Brando V, Maughan M (2010) Dispersal of suspended sediments and nutrients in the Great Barrier Reef lagoon during river discharge events: conclusions from satellite remote sensing and concurrent flood plume sampling. Mar Freshw Res 61:651–664
Bruno JF, Selig ER (2007). Regional decline of coral cover in the Indo-Pacific: timing, extent and subregional comparisons. PLoS ONE 2:e711
Caperon J, Cattell SA, Krasnick G (1971) Phytoplankton kinetics in a subtropical estuary: eutrophication. Limnol Oceanogr 16:599–607
Cooper TF, Uthicke S, Humphrey C, Fabricius KE (2007) Gradients in water column nutrients, sediment parameters, irradiance and coral reef development in the Whitsunday Region, central Great Barrier Reef. Estuar Coast Shelf Sci 74:458–470
Cooper TF, Ridd PV, Ulstrup KE, Humphrey C, Slivkoff M, Fabricius K (2008) Temporal dynamics in coral bioindicators for water quality on coastal coral reefs of the Great Barrier Reef. Mar Freshw Res 59(8):703–716
Cox EF, Ribes M, Kinzie RA (2006) Temporal and spatial scaling of planktonic responses to nutrient inputs into a subtropical embayment. Mar Ecol Prog Ser 324:19–35
Crosbie ND, Furnas MJ (2001) Abundance, distribution and flowcytometric characterization of picophytoprokaryote populations in central (17_S) and southern (20_S) shelf waters of the Great Barrier Reef. J Plankton Res 23:809–828
De’ath G, Fabricius KE (2008) Water quality of the Great Barrier Reef: distributions, effects on reef biota and trigger values for the conservation of ecosystem health. Research publication no. 89. Great Barrier Marine Park Authority, Report to the Great Barrier Reef Marine Park Authority and published by the Great Barrier Reef Marine Park Authority, Townsville, 104 pp
De’ath G, Fabricius KE (2010) Water quality as regional driver of coral biodiversity and macroalgal cover on the Great Barrier Reef. Ecol Appl 20:840–850
Delesalle B, Pichon M, Frankignoulle M, Gattuso J-P (1993) Effects of a cyclone on coral reef phytoplankton biomass, primary production and composition (Moorea Island, French Polynesia). J Plankton Res 15:1413–1423
Devantier LM, De’ath G, Turak E, Done TJ, Fabricius KE (2006) Species richness and community structure of reef-building corals on the nearshore Great Barrier Reef. Coral Reefs 25:329–340
Devlin M, Brodie J (2005) Terrestrial discharge into the Great Barrier Reef lagoon: nutrient behaviour in coastal waters. Mar Pollut Bull 51:9–22
Devlin M, Schaffelke B (2009) Extent and exposure of riverine flood plumes in the Tully coastal region. Mar Freshw Res 60:1109–1122
Devlin M, Waterhouse J, Taylor J, Brodie J (2001) Flood plumes in the Great Barrier Reef: spatial and temporal patterns in composition and distribution. Great Barrier Reef Marine Park Authority research publication no. 68. Great Barrier Reef Marine Park Authority, Townsville
Devlin MJ, Best M, Haynes D (eds) (2007) Implementation of the Water Framework Directive in European Waters (special edition). Mar Pollut Bull 55:1–6
Devlin M, Brodie J, Lewis S, Bainbridge Z (2009) Reef rescue marine monitoring program: flood plumes in the GBR—case studies for marine monitoring program, Tully and Burdekin. Final report for 2008/2009 activities JCU. ACTFR Catchment to Reef Group, James Cook University, Townsville. http://www.rrrc.org.au/mmp/mmp_pubs.html
Devlin M, Harkness P, Waterhouse J (2010) Mapping of risk and exposure of Great Barrier Reef ecosystems to anthropogenic water quality. A review and synthesis of current status. ACTFR report number 10/12. James Cook University
Diaz-Pulido G, McCook LJ, Dove S, Berkelmans R, Roff G, Kline DI, Weeks S, Evans RD, Williamson DH, Hoegh-Guldberg O (2009) Doom and boom on a resilient reef: climate change, algal overgrowth and coral recovery. PLoS One 4(4):e5239
Fabricius KE (2005) Effects of terrestrial runoff on the ecology of corals and coral reefs: Review and synthesis. Mar Pollut Bull 50:125–146
Fabricius KE (2007) Conceptual model of the effects of terrestrial runoff on the ecology of corals and coral reefs of the GBR. Report to the Australian Government’s Marine and Tropical Sciences Research Facility (MTSRF), 24 pp
Fabricius KE, De’ath G (2004) Identifying ecological change and its cause: a case study on coral reefs. Ecol Appl 14(5):1448–1465
Fabricius K, De’ath G, McCook L, Turak E, Williams D (2005) Changes in algal, coral and fish assemblages along water quality gradients on the inshore Great Barrier Reef. Mar Pollut Bull 51:384–398
Fabricius KE, Okaji K, De’ath G (2010) Three lines of evidence to link outbreaks of the crown-of-thornsseastar Acanthaster planci to the release of larval food limitation. Coral Reefs 29:593–605
Foden J, Devlin M, Malcolm SJ, Mills D (2010) Searching for undesirable disturbance: an application of the OSPAR eutrophication assessment method to marine waters of England and Wales. Biogeochemistry. doi: 10.1007/s10533-010-9475-9
Furnas MJ (2003) Catchments and corals: terrestrial runoff to the Great Barrier Reef. Australian Institute of Marine Science and Reef CRC, Townsville
Furnas MJ, Brodie J (1996) Current status of nutrient levels and other water quality parameters in the Great Barrier Reef. In: Hunter H, Eyles AG, Rayment GE (eds) Downstream effects of land use. Department of Natural Resources, Brisbane, pp 9–23
Furnas MJ, Mitchell AW (1986) Phytoplankton dynamics in the central Great Barrier Reef—I. Seasonal changes in biomass and community structure and their relations to intrusive activity. Cont Shelf Res 6:363–384
Furnas MJ, Mitchell AW, Skuza M (1995) Nitrogen and phosphorus budgets for the central Great Barrier Reef. Research report no. 36, Great Barrier Reef Marine Park Authority, Townsville
Furnas MJ, Mitchell A, Skuza M (1997) Shelf scale nitrogen and phosphorus budgets for the Central Great Barrier Reef (16°–19° S). In: Lessios HA, Macintyre IG (eds) Proceedings of the 8th international coral reef symposium, vol 1, Panama City, Panama, pp 809–814
Furnas MJ, Mitchell A, Skuza M, Brodie J (2005) The other 90 percent: phytoplankton responses to enhanced nutrient availability in the Great Barrier Reef lagoon. Mar Pollut Bull 51:253–265
Gagan MK, Sandstrom MW, Chivas AR (1987) Restricted terrestrial carbon input to the continental shelf during cyclone Winifred: implications for terrestrial run-off to the Great Barrier Reef province. Coral Reefs 6:113–119
Gilbert M, Brodie J (2001) Population, major land use in the Great Barrier Reef catchment area: spatial and temporal trends. Great Barrier Reef Marine Park Authority, Townsville
Gin KY-H, Lin X, Zhang S (2000) Dynamics and size structure of phytoplankton in the coastal waters of Singapore. J Plankton Res 22(8):1465–1484
Great Barrier Reef Marine Park Authority (2009) Water quality guidelines for the Great Barrier Reef Marine Park. Great Barrier Reef Marine Park Authority, Townsville, 99 pp
Grigg RW (1995) Coral reefs in an urban embayment in Hawaii: a complex case history controlled by natural and anthropogenic stress. Coral Reefs 14(4):253–266
Hallock P (1988) The role of nutrient availability in bioerosion: consequences to carbonate buildups. Palaeogeogr Palaeoclimatol Palaeoecol 63:275–291
Harris GP (2001) Biogeochemistry of nitrogen and phosphorus in Australian catchments, rivers and estuaries: effects of land use and flow regulation and comparisons with global patterns. Mar Freshw Res 52(1):139–149
Harrison PL, Ward S (2001) Elevated levels of nitrogen and phosphorus reduce fertilisation success of gametes from scleractinian reef corals. Mar Biol 139:1057–1068
Haynes D, Ralph P, Prange J, Dennison B (2000) The impact of the herbicide diuron on photosynthesis in three species of tropical seagrass. Mar Pollut Bull 41:288–293
Haynes D, Brodie J, Christie C, Devlin M, Michalek-Wagner K, Morris S, Ramsay M, Storrie J, Waterhouse J, Yorkston H (2001) Great Barrier Reef water quality current issues. Great Barrier Reef Marine Park Authority, Townsville
Haynes D, Brodie J, Waterhouse J, Bainbridge Z, Bass D, Hart B (2007) Assessment of the water quality and ecosystem health of the Great Barrier Reef (Australia): conceptual models. Environ Manage 40:993–1003
Hopcroft RR, Roff JC (2003) Response of tropical marine phytoplankton communities to manipulations of nutrient concentration and grazing pressure. Bull Mar Sci 73:397–420
Hughes TP, Szmant AM, Stenek R, Carpenter R, Miller S (1999) Algal blooms on coral reefs: what are the causes? Limnol Oceanogr 44:1583–1586
Hughes TP, Rodrigues MJ, Bellwood DR, Ceccerelli D, Hoegh-Guldberg O, McCook L, Moltchaniwskyj N, Pratchett MS, Steneck RS, Willis BL (2007) Regime-shifts, herbivory and the resilience of coral reefs to climate change. Curr Biol 17:360–365
Hunter CL, Evans CW (1995) Coral reefs in Kaneohe Bay, Hawaii: two centuries of western influence and two decades of data. Bull Mar Sci 57(2):501–515
Hunter HM, Walton RS (2008) Land-use effects on fluxes of suspended sediment, nitrogen and phosphorus from a river catchment of the Great Barrier Reef, Australia. J Hydrol 356:131–146
Hutchings P, Peyrot-Clausade M, Osnorno A (2005) Influence of land runoff on rates and agents of bioerosion of coral substrates. Mar Pollut Bull 51:438–447
Jacquet S, Delesalle B, Torréton J, Blanchot J (2006) Response of phytoplankton communities to increased anthropogenic influences (southwestern lagoon, New Caledonia). Mar Ecol Prog Ser 320:65–78
Johnson JE, Waterhouse J, Maynard JA, Morris S (Writing Team) (2010) Reef rescue marine monitoring program: 2008/2009 synthesis report. Report prepared by the Reef and Rainforest Research Centre Consortium of monitoring providers for the Great Barrier Reef Marine Park Authority. Reef and Rainforest Research Centre Limited, Cairns, 160 pp. http://www.rrrc.org.au/mmp/mmp_pubs.html
Jompa J, McCook LJ (2002) The effect of herbivory on competition between a macroalgae and a hard coral. J Exp Mar Biol Ecol 271:25–39
Jompa J, McCook LJ (2003) Contrasting effects of turf algae on corals: massive Porites spp. are unaffected by mixed-species turfs, but killed by the red alga Anotrichium tenue . Mar Ecol Prog Ser 258:29–86
Jupiter S, Roff G, Marion G, Henderson M, Schrameyer V, McCulloch M, Hoegh-Guldberg O (2008) Linkages between coral assemblages and coral proxies of terrestrial exposure along a cross-shelf gradient on the southern Great Barrier Reef. Coral Reefs 27:887–903
Kiene WE (1997) Enriched nutrients and their impact on bioerosion: results from ENCORE. In: Proceedings of the eighth international coral reef symposium
Kiene WE, Hutchings PA (1994) Bioerosion experiments at Lizard Island, Great Barrier Reef. Coral Reefs 13:91–98
Kinsey DW (1988) Coral reef system response to some natural and anthropogenic stresses. Galaxea 7:113–128
Kinsey DW (1991) Can we resolve the nutrient issue for the Reef? Search 22:119–121
Kline DI, Kuntz NM, Breitbart M, Knowlton N, Rohwer F (2006) Role of elevated organic carbon levels and microbial activity in coral mortality. Mar Ecol Prog Ser 314:119–125
Koop K, Booth D, Broadbent A, Brodie J, Bucher D, Capone D, Coll J, Dennison W, Erdmann M, Harrison P, Hoegh-Guldberg O, Hutchings P, Jones GB, Larkum AWD, O’Neil J, Steven A, Tentori E, Ward S, Williamson J, Yellowlees D (2001) ENCORE: the effect of nutrient enrichment on coral reefs. Synthesis of results and conclusions. Mar Pollut Bull 42:91–120
Lapointe BE (1997) Nutrient thresholds for bottom-up control of macroalgal blooms on coral reefs in Jamaica and southeast Florida. Limnol Oceanogr 42(5–2):1119–1131
Lapointe BE, Barile PJ, Yentsch CS, Littler MM, Littler DS, Kakuk B (2004) The relative importance of nutrient enrichment and herbivory on macroalgal communities near Norman’s Pond Cay, Exumas Cays, Bahamas: a “natural” enrichment experiment. J Exp Mar Biol Ecol 298(2):275–301
Laws EA, Allen CB (1996) Water quality in a subtropical embayment more than a decade after diversion of sewage discharges. Pac Sci 50(2):194–210
Lewis SE, Brodie J, Ledee E, Alewijnse M (2006) The spatial extent of delivery of terrestrial materials from the Burdekin region of the Great Barrier Reef lagoon. ACTFR report no. 06/02, Australian Centre for Tropical Freshwater Research, James Cook University, Townsville
Lewis SE, Brodie JE, Bainbridge ZT, Rohde KW, Davis AM, Masters BL, Maughan M, Devlin MJ, Mueller JF, Schaffelke B (2009) Herbicides: a new threat to the Great Barrier Reef. Environ Pollut 157:2470–2484
Littler MM, Littler DS (1984) Models of tropical reef, biogenesis: the contribution of algae. Prog Phycol Res 3:323–364
Littler MM, Littler DS, Brooks BL (2006) Harmful algae on tropical coral reefs: bottom-up eutrophication and top-down herbivory. Harmful Algae 5:1–23
Loya Y (1976) Recolonisation of Red Sea corals affected by natural catastrophes and man-made perturbations. Ecology 57:278–289
Loya Y, Lubinevsky H, Rosenfeld M, Kramarsky-Winter E (2004) Nutrient enrichment caused by in situ fish farms at Eilat, Red Sea is detrimental to coral reproduction. Mar Pollut Bull 49(4):344–353
Maughan M, Brodie JE (2009) Reef exposure to river-borne contaminants: a spatial model. Mar Freshw Res 60:1132–1140
McClanahan TR, Graham NAJ (2005) Recovery trajectories of coral reef fish assemblages within Kenyan marine protected areas. Mar Ecol Prog Ser 294:241–248
McCook LJ (1999) Macroalgae, nutrients and phase shifts on coral reefs: scientific issues and management consequences for the Great Barrier Reef. Coral Reefs 18(4):357–367
McCook LJ (2001) Competition between coral and algal turfs along a water quality gradient in the nearshore central Great Barrier Reef. Coral Reefs 19:419–425
McCook LJ, Jompa J, Diaz-Pulido G (2001) Competition between corals and algae on coral reefs: a review of evidence and mechanisms. Coral Reefs 19:400–417
McKenzie L, Unsworth R (2009) Reef rescue marine monitoring program: intertidal seagrass. Final report for the sampling period 1 September 2008 to 31 May 2009. Fisheries Queensland, Cairns. http://www.rrrc.org.au/mmp/mmp_pubs.html
McKergow L, Prosser IP, Hughes AO, Brodie JE (2005) Regional scale nutrient modelling: exports to the Great Barrier Reef World Heritage Area. Mar Pollut Bull 51:186–199
Mellors JE, Waycott M, Marsh H (2005) Variation in biogeochemical parameters across intertidal seagrass meadows in the central Great Barrier Reef region. Mar Pollut Bull 51:335–342
Mitchell AW, Furnas M (1997) Terrestrial inputs of nutrients and suspended sediments to the Great Barrier Reef lagoon. The Great Barrier Reef: science, use and management, a national conference. James Cook University, Townsville, pp 59–71
Mitchell AW, Bramley RGV, Johnson AKL (1997) Export of nutrients and suspended sediment during a cyclone-mediated flood event in the Herbert River catchment, Australia. Mar Freshw Res 48:79–88
Mitchell A, Reghenzani J, Furnas M (2001) Nitrogen levels in the Tully River—a long-term view. Water Sci Technol 43:99–105
Mitchell C, Brodie J, White I (2005) Sediments, nutrients and pesticide residues in event flow conditions in streams of the Mackay Whitsunday Region, Australia. Mar Pollut Bull 51:23–36
Mitchell A, Reghenzani J, Faithful J, Furnas M, Brodie J (2009) Relationships between land use and nutrient concentrations in streams draining a ‘wet-tropics’ catchment in northern Australia. Mar Freshw Res 60:1097–1108
Moran PJ, De’ath G (1992) Estimates of the abundance of the crown-of-throns starfish Acanthaster planci in outbreaking and non-outbreaking populations on reefs within the Great Barrier Reef. Mar Biol 113:509–515. doi: 10.1007/BF00349178
Packett R, Dougall C, Rohde K, Noble R (2009) Agricultural lands are hot-spots for annual runoff polluting the southern Great Barrier Reef lagoon. Mar Pollut Bull 58:976–985
Pandolfi JM, Bradbury RH, Sala E, Hughes TP et al (2003) Global trajectories of the long-term decline of coral reef ecosystems. Science 301:955–958
Queensland Department of Premier and Cabinet (2009) Reef Water Quality Protection Plan 2009. For the Great Barrier Reef World Heritage Area and adjacent catchments. Queensland Department of Premier and Cabinet, Brisbane. http://www.reefplan.qld.gov.au/about/rwqpp.shtm
Rabalais NN (2002) Nitrogen in aquatic ecosystems. Ambio 31(2):102–112
Rayment GE (2003) Water quality in sugar catchments in Queensland. Water Sci Technol 48(7):35–47
Risk MJ, Sammarco PW, Edinger EN (1995) Bioerosion in Acropora across the continental shelf of the Great Barrier Reef. Coral Reefs 14:79–86
Rodier M, Le Borgne R (2008) Population dynamics and environmental conditions affecting Trichodesmium spp. (filamentous cyanobacteria) blooms in the south–west lagoon of New Caledonia. J Exp Mar Biol Ecol 358:20–32
Rohde K, Masters B, Fries N, Noble R, Carroll C (2008) Fresh and marine water quality in the Mackay Whitsunday region 2004–05 to 2006–07. Queensland Department of Natural Resources and Water for the Mackay Whitsunday Natural Resource Management Group, Australia
Sammarco PW, Risk M (1990) Large-scale patterns in internal bioerosion of Porites: cross continental shelf trends on the Great Barrier Reef. Mar Ecol Prog Ser 59:145–156
Schaffelke B, Thompson A, Carleton J, Davidson J, Doyle J, Furnas M, Gunn K, Skuza M, Wright M, Zagorskis I (2009) Reef rescue marine monitoring program. Final report of AIMS activities 2008/2009. Report submitted to the Reef and Rainforest Research Centre. Australian Institute of Marine Science, Townsville. http://www.rrrc.org.au/mmp/mmp_pubs.html
Shaw EC, Gabric AJ, McTainsh GH (2008) Impacts of Aeolian dust deposition on phytoplankton dynamics in Queensland coastal waters. Mar Freshw Res 59(11):951–962. doi: 10.1071/MF08087
Smith VS, Kimmerer WJ, Laws EA, Brock RE, Walsh TW (1981) Kaneohe Bay sewage diversion experiment: perspectives on ecosystem responses to nutritional perturbation. Pac Sci 35:279–340
Smith JE, Smith CM, Hunter CL (2001) An experimental analysis of the effects of herbivory and nutrient enrichment on benthic community dynamics on a Hawaiian reef. Coral Reefs 19:332–342
Smith JE, Shaw M, Edwards RA, Obura D, Pantos O et al (2006) Indirect effects of algae on coral: algae-mediated, microbe-induced coral mortality. Ecol Lett 9:835–845
Sotka EE, Hay ME (2009) Effects of herbivores, nutrient enrichment, and their interactions on macroalgal proliferation and coral growth. Coral Reefs 28:555–568
Stimson J, Conklin E (2008) Potential reversal of a phase shift: the rapid decrease in the cover of the invasive green macroalga Dictyosphaeria cavernosa (Forsskål) on coral reefs in Kaneohe Bay, Oahu, Hawaii. Coral Reefs 27:717–726
Stimson J, Larned ST, Conklin E (2001) Effects of herbivory, nutrient levels, and introduced algae on the distribution and abundance of the invasive macroalga Dictyosphaeria cavernosa in Kaneohe Bay, Hawaii. Coral Reefs 19:343–357
Sweatman H, Burgess S, Cheal AJ, Coleman G, Delean S, Emslie M, Miller I, Osborne K, McDonald A, Thompson A (2005) Long-term monitoring of the Great Barrier Reef. Status report no. 7. CDRom. Australian Institute of Marine Science, Townsville
Sweatman HPA, Cheal AJ, Coleman GJ, Emslie MJ, Johns K, Jonker M, Miller IR, Osborne K (2008) Long-term Monitoring of the Great Barrier reef, Status Report. 8. Australian Institute of Marine Science, 369 pp
Tada K, Sakai K, Nakano Y, Takemura A, Montani S (2003) Size-fractionated phytoplankton biomass in coral reef waters off Sesoko Island, Okinawa, Japan. J Plankton Res 25(8):991–997
Tenório MMB, Le Borgne R, Rodier M, Neveux J (2005) The impact of terrigeneous inputs on the Bay of Ouinné (New Caledonia) phytoplankton communities: a spectrofluorometric and microscopic approach. Estuar Coast Shelf Sci 64:531–545
Tett P, Gowen R, Mills D, Fernandes T, Gilpin L, Huxham M, Kennington K, Read P, Service M, Wilkinson M, Malcolm S (2007) Defining and detecting undesirable disturbance in the context of eutrophication. Mar Pollut Bull 53:282–297
Thompson A, Schaffelke B, De’ath G, Cripps E, Sweatman H (2010) Water quality and ecosystem monitoring programme-reef water quality protection plan. Synthesis and spatial analysis of inshore monitoring data 2005-08. Report to the Great Barrier Reef Marine Park Authority. Australian Institute of Marine Science, Townsville, 78 pp. http://www.rrrc.org.au/mmp/mmp_pubs.html
Tomascik T, Sander F (1985) Effects of eutrophication on reef-building corals. I. Growth rates of the reef-building coral Montastera annularis . Mar Biol 87:143–155
Touchette BW, Burkholder JM (2000) Overview of the physiological ecology of carbon metabolism in seagrasses. J Exp Mar Biol Ecol 250:169–205
Tribollet A, Golubic S (2005) Cross-shelf differences in the pattern and pace of bioerosion of experimental carbonate substrates exposed for 3 years on the northern Great Barrier Reef, Australia. Coral Reefs 24:422–434
Udy JW, Dennison WC, Lee Long W, McKenzie LJ (1999) Responses of seagrass to nutrients in the Great Barrier Reef, Australia. Mar Ecol Prog Ser 185:257–271
Van Duyl FC, Gast GJ, Steinhoff W, Kloff S, Veldhius MJW, Bak RPM (2002) Factors influencing the short-term variation in phytoplankton composition and biomass in coral reef waters. Coral Reefs 21:293–306
van Woesik R, Tomascik T, Blake S (1999) Coral assemblages and physico-chemical characteristics of the Whitsunday Islands: evidence of recent community changes. Mar Freshw Res 50:427–440
Walker TA (1991) Is the Reef really suffering from chronic pollution? Search 22:115–117
Walker TA, O’Donnell G (1981) Observations on nitrate, phosphate and silicate in Cleveland Bay, Northern Queensland. Aust J Mar Freshw Res 32:877–887
Walker D, Dennison W, Edgar G (1999) Status of Australian seagrass research and knowledge, Chap 1. In: Walker D, Dennison W, Edgar G (eds) Seagrass in Australia. CSIRO Australia, Collingwood
Wallace J, Stewart L, Hawdon A, Keen R (2008) The role of coastal floodplains in generating sediment, nutrient fluxes to the Great Barrier Reef Lagoon in Australia. Ecohydrological processes, sustainable floodplain management opportunities, concepts for water hazard mitigation, ecological, socio-economic sustainability. 19–23 May 2008 Lodz, Poland. Int J Ecohydrol Hydrobiol 8:183–194
Wallace J, Stewart L, Hawdon A, Keen R, Karim F, Kemei J (2009) Flood water quality and marine sediment and nutrient loads from the Tully and Murray catchments in north Queensland, Australia. Mar Freshw Res 60:1123–1131
Ward S, Harrison PL (1997) The effect of elevated nutrient levels on the settlement of coral larvae during the ENCORE experiment, Great Barrier Reef, Australia. In: Lessios HA, McIntyre IG (eds) Proceedings of the eighth international coral reef symposium, pp 890–896
Ward S, Harrison PL (2000) Changes in gametogenic patterns and fecundity of acroporid reef corals that were exposed to elevated nitrogen and phosphorus during the ENCORE experiment. J Exp Mar Biol Ecol 246:179–221
Waterhouse J, Grundy M, Brodie J, Gordon I, Yorkston H, Eberhard R (2009) Flagship basin study—Great Barrier Reef. In: Ferrier R, Jenkins J (eds) Handbook of catchment management. Blackwell Publishing, United Kingdom
Wilkinson C (2008) Status of coral reefs of the world: 2008. Global Coral Reef Monitoring Network and Reef and Rainforest Research Centre, Townsville
Wismer S, Hoey AS, Bellwood DR (2009) Cross-shelf benthic community structure on the Great Barrier Reef: relationships between macroalgal cover and herbivore biomass. Mar Ecol Prog Ser 376:45–54
Wooldridge SA (2009a) A new conceptual model for the warm-water breakdown of the coral-algae endosymbiosis. Mar Freshw Res 60:483–496
Wooldridge SA (2009b) Water quality and coral bleaching thresholds: Formalising the linkage for the inshore reefs of the Great Barrier Reef, Australia. Mar Pollut Bull 58:745–751
Wooldridge SA, Done TJ (2009) Improved water quality can ameliorate effects of climate change on corals. Ecol Appl 19:1492–1499
Download references
Author information
Present address: SPREP (Secretariat of the Pacific Regional Environment Programme), P.O. Box 240, Apia, Samoa
Authors and Affiliations
Catchment to Reef Research Group, Australian Centre for Tropical Freshwater Research, James Cook University, Townsville, 4811, Australia
J. E. Brodie, M. Devlin & J. Waterhouse
Great Barrier Reef Marine Park Authority, P.O. Box 1379, Townsville, 4810, Australia
You can also search for this author in PubMed Google Scholar
Corresponding author
Correspondence to J. E. Brodie .
Rights and permissions
Reprints and permissions
About this article
Brodie, J.E., Devlin, M., Haynes, D. et al. Assessment of the eutrophication status of the Great Barrier Reef lagoon (Australia). Biogeochemistry 106 , 281–302 (2011). https://doi.org/10.1007/s10533-010-9542-2
Download citation
Received : 17 November 2009
Accepted : 22 October 2010
Published : 17 November 2010
Issue Date : November 2011
DOI : https://doi.org/10.1007/s10533-010-9542-2
Share this article
Anyone you share the following link with will be able to read this content:
Sorry, a shareable link is not currently available for this article.
Provided by the Springer Nature SharedIt content-sharing initiative
- Eutrophication
- Great Barrier Reef
- Water quality management
- Find a journal
- Publish with us
- Track your research
- View source
- View history
- Community portal
- Recent changes
- Random page
- Featured content
- What links here
- Related changes
- Special pages
- Printable version
- Permanent link
- Page information
- Browse properties
Case studies eutrophication
- 1.1 Introduction
- 1.2 Causes and consequences
- 1.3 Solutions
- 2 Human health impacts of eutrophication (Case study: Humans at the top of the food web)
- 3 Economic impacts of eutrophication (Case study: Shellfish flavour)
- 4 Recreational and aesthetic impacts of eutrophication (Case study: Foam on the beach)
- 5 References
Ecological impacts of eutrophication (Case study: Eutrophication and dead zones)

Introduction
Dead zones are very low oxygen areas ( hypoxic ) in the ocean where marine life including fish, crabs and clams cannot survive. In the 1970s oceanographers began noting increased instances of dead zones. A 2008 study counted 405 dead zones worldwide [1] . Hypoxia is a natural phenomenon that occurs periodically in coastal waters around the world. During the last 50 years however, increases in key pollutants from human activities on land have thrown many coastal ecosystems out of balance, resulting in expanded dead zone regions.
Causes and consequences
Aquatic and marine dead zones can be caused by an increase in nutrients (mainly nitrates and phosphates ) in the water known as eutrophication . Major nutrient sources come from human activities such as the use of fertilizers in agriculture and the burning of fossil fuels. These nutrients lead to a rapid increase of the density of certain types of phytoplankton resulting in algal blooms . The organic matter produced by these phytoplankton species at the surface of the ocean sinks to the bottom (the benthic zone) where bacteria break it down. The bacteria use oxygen and give off carbon dioxide during this breakdown. Fish and mobile invertebrates can migrate out of hypoxic areas. Plants and animals that are slow moving or attached to the bottom (sea grass, worms and clams) cannot escape from the dangers of hypoxic waters and will die. The dead zone of the Baltic Sea is probably the largest worldwide [1] . Overfishing of Baltic cod has greatly intensified the problem. Cod eat sprats, a small, herring-like species that eat microscopic zooplankton that in turn eat the algae. So, fewer cods and an explosion of zooplankton-eating sprats means more algae and less oxygen- a vicious cycle develops [2] .
The main goal in reducing dead zones is to keep fertilizers on the land and out of coastal waters. The Black Sea dead zone largely disappeared between 1991 and 2001 after fertilizers became too costly to use following the collapse of the Sovjet Union and the demise of eastern European economies. Nutrients loads entering the sea where therefore considerably reduced. Fishing has again become a major economic activity in the region. However, our ocean ecosystems are fragile and the combined threats of climate change, overexploitation, pollution and habitat loss,all mostly caused by human activity, are undermining the sustainability. Expanded dead zones caused by global warming will remain for thousands of years and have harmful long-term effects on ocean ecosystems.
Human health impacts of eutrophication (Case study: Humans at the top of the food web)

The consumption of shellfish (e.g. mussels, clams) is one of the most common ways for algal toxins to impact human health. Marketable shellfish are generally considered to be safe, but in spite of these precautions, there are known illnesses. One dramatic incident occurred in 1990 when six fishermen almost died from eating mussels during a fishing trip on Georges Bank, a productive offshore finfish and shellfish area. The fishermen became ill after eating a pot of mussels they had caught in their nets. The Captain, who had joined the meal later than the rest of the crew, witnessed his fellow fishermen become incapacitated due to the paralytic effects of the toxin. He himself also became ill, but was capable of sending an urgent radio message to the US Coast Guard. In the hospital they were treated using respiratory therapy to sustain their breathing and prevent them from dying due to paralysis of the lungs. The event, presumably caused by a massive Alexandrium [3] bloom transported offshore from areas along the northeast coast, closed the surf clam industry on Georges Bank to further harvest. Source: WHOI [4]
Economic impacts of eutrophication (Case study: Shellfish flavour)
Some algae and diatoms impart off-flavours or bitter taints to shellfish, rendering them unpalatable and unmarketable. In 1987 in Port Phillip Bay, Melbourne, Australia, a bloom of the diatom Rhizosolenia chunii [5] [6] occurred and 3 species of shellfish within the bay, mussels, oysters and scallops, developed a powerful bitter taint. The taint was so persistent and unpleasant that the mussels from the bay were unmarketable for 7 months, causing a revenue loss of approximately $1 million.
Recreational and aesthetic impacts of eutrophication (Case study: Foam on the beach)

Some algae, particularly of the taxa Phaeocystis [7] , produce a mucus, which when disturbed produce a foam. These algae are more prone to develop when there is little competition. It seems that in areas such as the south-east coast of the North sea, where all the silica has been captured by diatoms in estuarine regions, the residual nitrogen is used by Phaeocystis to bloom. They produce large amount of mucus which, if the weather is windy, will in turn be transformed into large amounts of foam covering extensive areas of beach and lake shores. Besides the impact on the landscape and the nuisance it represents for tourists, this foam is suspected of disturbing flat fish larvae development. This phenomenon is frequently observed at the Belgian and Dutch coasts, and appears from time to time in Germany.
Coastal Wiki articles on eutrophication are listed in the category Eutrophication .
- ↑ Diaz R. J, Rosenberg R. (2008): Spreading dead zones and consequences for marine ecosystems. Science 321, 629.
- ↑ Westman, 2010; cited in Owen 2010. World's Largest Dead Zone Suffocating Sea, National Geographic News.
- ↑ WoRMS (2012). Alexandrium Halim, 1960 emend. Balech, 1989. In: Guiry, M.D. & Guiry, G.M. (2012). AlgaeBase. World-wide electronic publication, National University of Ireland, Galway. Accessed through: World Register of Marine Species at http://www.marinespecies.org/aphia.php?p=taxdetails&id=109470
- ↑ http://www.whoi.edu/science/B/redtide/foodweb/shellwedolunch.html
- ↑ Kraberg, A. (2011). Rhizosolenia chunii Karsten, 1905. Accessed through: World Register of Marine Species at http://www.marinespecies.org/aphia.php?p=taxdetails&id=341502
- ↑ Parry, G.D., Langdon, J.S. & Huisman, J.M. (1989). Toxic effects of a bloom of the diatom Rhizosolenia chunii on shellfish in Port Phillip Bay, southeastern Australia. Marine Biology, Berlin 102: 25-41.
- ↑ Guiry, M.D. (2011). Phaeocystis. In: Guiry, M.D. & Guiry, G.M. (2011). AlgaeBase. World-wide electronic publication, National University of Ireland, Galway. Accessed through: World Register of Marine Species at http://www.marinespecies.org/aphia.php?p=taxdetails&id=115088
- Articles by Knockaert, Carolien
- Eutrophication
- This page was last edited on 16 February 2024, at 12:17.
- Privacy policy
- About Coastal Wiki
- Disclaimers

- Azerbaijani
- Chinese (Simplified)
- Chinese (Traditional)
- Haitian Creole
- Kinyarwanda
- Kurdish (Kurmanji)
- Kurdish (Soranî)
- Odia (Oriya)
- Scots Gaelic
Eutrophication (nutrient enrichment)
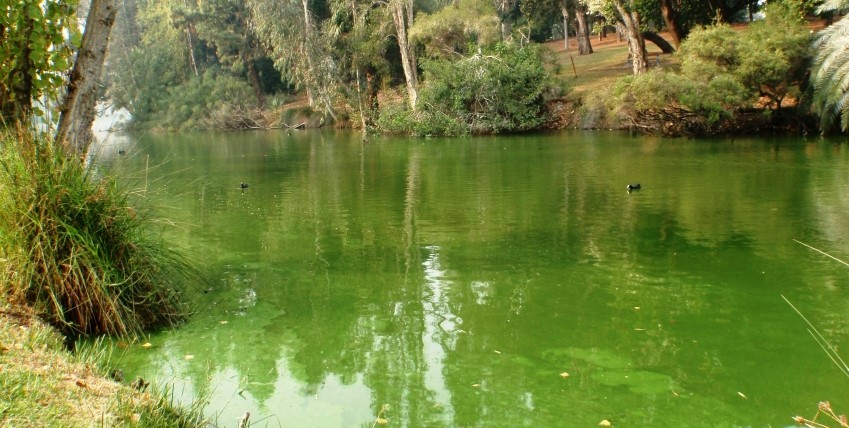
Eutrophication means nutrient enrichment. It causes excess primary productivity (algal overgrowth) in waterways, resulting in poor water quality and reduced amenity, and in the worst cases, algal blooms, low oxygen and fish deaths.
Eutrophication can occur naturally but is a widespread problem as a result of human activities.
Nutrients are essential for the growth and survival of algae and aquatic plants, just as they are for terrestrial (land) plants. However, only small amounts of each nutrient are required in a natural ecosystem and any additional increase of these nutrients in waterways can quickly become a problem.
Why are there excess nutrients in our waterways?
Human activities have altered the way water moves through the landscape.
By clearing vegetation for agriculture or urban development, and by constructing drainage, water is moved more quickly off the catchment and into receiving waterways and water bodies, carrying with it excess sediment and nutrients.
This problem is exacerbated when extra nutrients are applied to the catchment in the form of fertiliser and animal manure, sewage, detergents or industrial effluent, and also by changing the types of plants present.
Where water passes slowly overland through vegetation or slowly through vegetated wetlands, rivers and estuaries, some of the sediment and nutrients can be trapped and absorbed.
However, all too often the fringing riparian vegetation around rivers and estuaries has also been removed or degraded, or water is moved rapidly through drainage channels, and there is insufficient opportunity for the removal of sediment and nutrients before they enter receiving waterways.
Compounds that contain nitrogen and phosphorus are the most common culprits causing eutrophication. In general these nutrients are not toxic at the concentrations typically found in nature, however they can have a large impact on the health of rivers and estuaries.
How does it impact our waterways?
High concentrations of nutrients encourage excessive algal growth and may result in nuisance or toxic algal blooms . Eventually when these blooms collapse and die, the resulting decomposition of the algal cells by bacteria removes oxygen from the water, sometimes causing mass fish kill events .
Nutrients are also temporarily stored in river and estuary sediments , but under certain environmental conditions, such as when there is low oxygen, the nutrients can re-enter the overlying water and be available again to grow more algae.
Eutrophication can stimulate the growth of algae to the extent that they begin to dominate an aquatic system, often to the exclusion of other species. Affected ecosystems lose their biodiversity, typically containing high populations of only a few plant species. Once simplified ecosystems occur, the natural cycling of nutrients in the system is interrupted and problems become persistent and recurring.
Eutrophication and algal overgrowth reduce water quality and affect the recreational use and enjoyment of our waterways. Common consequences are unpleasant odours, excessive nuisance insect production (e.g. mosquitoes and midges) and making them less suitable for swimming, boating and fishing.
Excess nutrients in run-off from fertiliser and effluent on agricultural land is the largest source of nutrients entering estuaries in south-west catchments.
Our Healthy Estuaries WA program works with catchment and industry groups and farmers to reduce the nutrient run-off from farms, improve water quality, and reduce incidents of algal blooms, low oxygen and fish deaths.
We assess the concentrations of nutrients at a range of sites in our south-west rivers and estuaries, stormwater drains and groundwater resources. Understanding the concentrations of nutrients both within and entering our rivers and estuaries helps us assess the health of waterways and determine management solutions to minimise the occurrence of algal blooms and fish kill events. This information also supports decisions about where to apply remediation (such as oxygenation ) or undertake riparian revegetation to improve water quality.
Long term monitoring allows us to assess trends and report whether nutrient conditions are improving or degrading - for examples, see catchment nutrient reports , river water quality assessment and estuary water quality assessment .
We can also use this data and our knowledge of land use to construct catchment models, which help us predict where nutrients are being exported from the landscape. These models inform our water quality improvement plans for priority estuaries within our Healthy Estuaries WA program.
Resources and publications
The water quality information our department and other organisations collect is available from our Water Information Reporting portal.
For more information refer to our water quality page.
Technical guidance about water quality sampling and program design see:
- Field sampling guidelines: A guideline for field sampling for surface water quality monitoring programs
- Water quality monitoring program design: A guideline to the development of surface water quality monitoring programs
For more information about the Swan and Canning Rivers see:
- Department of Biodiversity, Conservation and Attractions webpage Waterway health in the Swan Canning Riverpark
- River Science 5: Sources of nutrients to the Swan and Canning rivers
High rainfall pastures:
- Environmental impact of nitrogen and phosphorus fertilisers in high rainfall areas of Western Australia (Department of Primary Industries and Regional Development)
Related services and information
- Public drinking water source areas
- Gingin groundwater allocation plan
- Considering waterways in planning and development
- Gascoyne River flow advice
- Shallow Groundwater Systems investigations – Perth
- Strategies to rebalance Gnangara - Exciting finds in science
- South West groundwater areas allocation plan
- Drinking water source protection reports - C to D
Related pages
- Threats to our waterways
Provided by
- Facebook share (Opens in a new tab/window)
- Twitter (Opens in a new tab/window)
- LinkedIn (Opens in a new tab/window)
Acknowledgement of Country
The Government of Western Australia acknowledges the traditional custodians throughout Western Australia and their continuing connection to the land, waters and community. We pay our respects to all members of the Aboriginal communities and their cultures; and to Elders both past and present.

An official website of the United States government
The .gov means it’s official. Federal government websites often end in .gov or .mil. Before sharing sensitive information, make sure you’re on a federal government site.
The site is secure. The https:// ensures that you are connecting to the official website and that any information you provide is encrypted and transmitted securely.
- Publications
- Account settings
Preview improvements coming to the PMC website in October 2024. Learn More or Try it out now .
- Advanced Search
- Journal List
- J Zhejiang Univ Sci B
- v.9(3); 2008 Mar
Mechanisms and assessment of water eutrophication *
Xiao-e yang.
1 MOE Key Laboratory of Polluted Environment Remediation and Ecological Health, Zhejiang University, Hangzhou 310029, China
2 Zhejiang Provincial Key Laboratory of Subtropic Soil and Plant Nutrition, Zhejiang University, Hangzhou 310029, China
3 Institute of Food and Agricultural Sciences, Indian River Research and Education Center, University of Florida, Fort Pierce, FL 34945, USA
Water eutrophication has become a worldwide environmental problem in recent years, and understanding the mechanisms of water eutrophication will help for prevention and remediation of water eutrophication. In this paper, recent advances in current status and major mechanisms of water eutrophication, assessment and evaluation criteria, and the influencing factors were reviewed. Water eutrophication in lakes, reservoirs, estuaries and rivers is widespread all over the world and the severity is increasing, especially in the developing countries like China. The assessment of water eutrophication has been advanced from simple individual parameters like total phosphorus, total nitrogen, etc., to comprehensive indexes like total nutrient status index. The major influencing factors on water eutrophication include nutrient enrichment, hydrodynamics, environmental factors such as temperature, salinity, carbon dioxide, element balance, etc., and microbial and biodiversity. The occurrence of water eutrophication is actually a complex function of all the possible influencing factors. The mechanisms of algal blooming are not fully understood and need to be further investigated.
INTRODUCTION
Water eutrophication is one of the most challenging environmental problems in the world. The increasing severity of water eutrophication has been brought to the attention of both the governments and the public in recent years. The mechanisms of water eutrophication are not fully understood, but excessive nutrient loading into surface water system is considered to be one of the major factors (Fang et al., 2004 ; Tong et al., 2003 ). The nutrient level of many lakes and rivers has increased dramatically over the past 50 years in response to increased discharge of domestic wastes and non-point pollution from agricultural practices and urban development (Mainstone and Parr, 2002 ). For more than 30 years, nutrient enrichment, especially phosphorus (P) and nitrogen (N), has been considered as a major threat to the health of coastal marine waters (Andersen et al., 2004 ). Once a water body is eutrophicated, it will lose its primary functions and subsequently influence sustainable development of economy and society. Therefore, nowadays the solution of water eutrophication and recovery of the multiple functions of the water system have become the key issues for environmental biologists. The main purpose of this paper is to provide a brief review on recent advances on understanding the mechanisms of water eutrophication and progresses in identifying the influence factors inducing water eutrophication.
DEFINITION AND OCCURRENCE OF WATER EUTROPHICATION
Definition of water eutrophication.
Lakes and estuaries accumulating large amounts of plant nutrients are called “eutrophic” (from the Greek words eu meaning “well” and trophe meaning “nourishment”). Eutrophication can be defined as the sum of the effects of the excessive growth of phytoplanktons leading to imbalanced primary and secondary productivity and a faster rate of succession from existence to higher serial stage, as caused by nutrient enrichment through runoffs that carry down overused fertilizers from agroecosystems and/or discharged human waste from settlements (Khan and Ansari, 2005 ). Water eutrophication can be greatly accelerated by human activities that increase the rate of nutrient input in a water body, due to rapid urbanization, industrialization and intensifying agricultural production. For lake aquatic ecosystems, human activities in the watershed can lead to loss of dominant species and functional groups, high nutrient turnover, low resistance, high porosity of nutrients and sediments, and the loss of productivity (Liu and Qiu, 2007 ). For example, aquaculture is one of many human activities contributing to the environmental decline of coastal waters and the collapse of fisheries stocks worldwide (Alongi et al., 2003 ). Because the influence of the human activities, excessive nitrogen, phosphorus and other nutrients are loaded into water bodies like lake, reservoirs, embouchure and bay, which could cause negative ecological consequences on aquatic ecosystem structures, processes and functions, result in the fast growth of algae and other plankton, and deteriorate water quality (Western, 2001 ). Generally speaking, water eutrophication is caused by the autotrophy algae blooming in water, which composes its bioplasm by sunlight energy and inorganic substances through photosynthesis—the process of eutrophication is described as follows:
According to above equation, it can be concluded that inorganic nitrogen and phosphorus are the major control factors for the propagation of algae, especially phosphorus. The Florida Everglades, a wetland of international importance, has been undergoing a significant shift in its native flora and fauna due to excessive total phosphorus (TP) loadings (an average of 147 ton per annum from 1995 to 2004) and an elevated mean TP concentration (69 μg/L of TP in 2004) from agricultural runoff and Lake Okeechobee outflow despite the use of over 17 000 ha of stormwater treatment areas (Richardson et al., 2007 ).
Assessment of water eutrophication
Surface water quality guidelines have been improved in recent years. The parameters to assess the ambient surface water quality have been increased. In China, the parameters for assessing environmental quality of surface water have been increased to over 30 (CNEPA, 2002 ). Five classes of surface water quality have been set up, and some selected parameters for assessing water quality of lakes or reservoirs are shown in Table Table1. 1 . However, there are no perfect evaluation criteria for assessing water eutrophication. Generally, the physical and chemical evaluation parameters were used to assess water eutrophication, mainly nutrient concentration (N and P), algal chlorophyll, water transparency and dissolved oxygen. Although there are many different assessment parameters, the concentrations of total nitrogen and phosphorus are the two basic ones. Cheng and Li ( 2006 ) used total nutrient status index (TNI) to assess eutrophication status of lakes. The calculation of total nutrient status index is as follows:
where, TNI is the sum of indexes of all nutrient parameters, TNI j is the TNI of j parameter, W j is the proportion of j parameter in the TNI, and r ij is the relation of chlorophyll a (Chla) to other parameters. The available parameters concerned include total nitrogen (TN), total phosphorus (TP), Chla, dissolved oxygen (DO), chemical oxygen demand by K 2 MnO 4 oxidation method (COD Mn ), biological oxygen demand (BOD 5 ), etc., and TN, TP and Chla are selected for calculating the TNI (Cheng and Li, 2006 ). Table Table2 2 shows the burthen values of TN, TP and TNI in various eutrophicated water. It has been shown that the eutrophication or red tide occurs when N concentration in water reaches 300 μg/L and P concentration reaches 20 μg/L. Richardson et al.( 2007 ) reported that exceeding a surface water mean TP threshold concentration of 15 μg/L causes an ecological imbalance in algal, macrophyte and macroinvertebrate assemblages as well as slough community structure in the Everglades areas. Therefore, it is considered that a threshold zone (12~15 μg/L) of TP may be more realistic and protective for all trophic levels.
The criteria of surface water quality for lakes or reservoir (CNEPA, 2002 )
DO: dissolved-oxygen; COD Mn : Chemical oxygen demand by K 2 MnO 4 oxidation method; COD Cr : Chemical oxygen demand by chromium oxidation method; BOD 5 : Biological oxygen demand; TN: Total nitrogen; TP: Total phosphorus
The burden values of N and P in various eutrophicated water
TN: Total nitrogen; TP: Total phosphorus, TNI: Total nutrient status index
Inglett and Reddy ( 2006 ) reported evidences to support the use of stable C (delta C-13) and N (delta N-15) isotopic ratios as indicators for eutrophication and shifts between N and P limitation. Lin et al.( 2006 ) compared the stable isotopes from dissolved nutrients and plants and water column nutrient parameters and integration of multiple proxies in a sediment core from Meiliang Bay of Taihu Lake, and found that differences in aquatic plant species and trophic status between East Taihu Bay and Meiliang Bay are indicated by their variations in delta C-13 and delta N-15 of aquatic plants and delta N-15 of NH 4 + -N. A significant influence of external nutrient inputs on water quality of Meiliang Bay is reflected in temporal changes in delta N-15 of NH 4 + -N and hydro-environmental parameters. The synchronous change between delta C-13 and delta N-15 values of sedimented organic matter (OM) has been attributed to elevated primary production at the beginning of eutrophication between 1950 and 1990, and then recent inverse correlation between them has been caused by the uptake of N-15-enriched inorganic nitrogen by phytoplankton grown under eutrophication and subsequent OM decomposition and denitrification in surface sediments, indicating that the lake has suffered from progressive eutrophication since 1990.
More sensitive biological indicators for assessing water eutrophication are needed to further study. Water eutrophication caused a degradation of healthy aquatic ecosystem, so the assessment methods and parameters should reflect the extents of aquatic ecosystem health. A set of ecological indicators including structural, functional and system-level aspects were proposed for a lake ecosystem health assessment, according to the structural, functional and system-level responses of lake ecosystems to chemical stresses including acidification, eutrophication, and copper, oil and pesticide contamination. The structural indicators included phytoplankton cell size and biomass, zooplankton body size and biomass, species diversity, macro- and micro-zooplankton biomass, the zooplankton/phytoplankton ratio, and the macrozooplankton/microzooplankton ratio. The functional indicators encompassed the algal C assimilation ratio, resource use efficiency, community production, gross production/respiration (i.e., P/R) ratio, gross production/standing crop biomass (i.e., P/B) ratio, and standing crop biomass/unit energy flow (i.e., B/E) ratio. The ecosystem-level indicators consisted of ecological buffer capacities, energy, and structural energy. Based on these indicators, a direct measurement method (DMM) and an ecological modeling method (EMM) for lake ecosystem health assessment were developed (Xu et al., 2001 ). The results of a case study demonstrate that both methods provided similar results which corresponded with the lake’s actual trophic state.
Occurrence of water eutrophication
The investigation from the UNEP (United Nation Environmental Protection) indicates that about 30%~40% of the lakes and reservoirs have been affected more or less by water eutrophication all over the world. Table Table3 3 cites selected samples of water eutrophication occurrence in lake, reservoir, estuary and river in the world. Erie Lake is excessively rich in nutrients (Reutter, 1989 ), which has resulted in huge blooms of floating blue-green algae and the attached green alga, Cladophora spp. These blooms have rolled onto beaches in large mats resembling green steel wool. Water eutrophication has been reported in USA for Washington Lake (Welch and Crooke, 1987 ), Okeechobee Lake (Schelske, 1989 ), City Park Lake (Ruley and Rusch, 2002 ), etc. In Lugano Lake, between Italy and Switzerland, a faster rate of eutrophication was reported due to excessive discharges from human settlements around the lake, owing to population increase and immigration (Barbieri and Simona, 2001 ). The majority of Danish lakes are highly eutrophic due to high nutrient input from domestic sources and agricultural activities (Jeppesen et al., 1999 ). Garg et al.( 2002 ) studied three lakes of Bhopal (Upper Lake, Lower Lake and Mansarovar Lake) in India, to assess the potential fertility of lentic waters and analyze the floral ecology. The highest level of eutrophication was found in Mansarovar Lake. The nutrient loading into the lake initially promoted the growth of phytoplanktons. Eutrophication constitutes a serious threat to many European lakes (Søndergaard et al., 2007 ), such as Pamvotis Lake in Northwest Greece (Romero et al., 2002 ), which has undergone cultural eutrophication over the past 40 years and is currently eutrophic. In South Africa, de Villiers ( 2007 ) reported that hypertrophic conditions indicated by TP levels prevail at least episodically at all of the Berg River monitoring stations; additionally, river water phosphate levels show a dramatic increase by a factor of more than 10 over the past 20 years, mainly due to anthropogenic inputs. Chivero Lake, Zimbabwe was reported to be hypertrophic and not sustainable (Nhapi, 2004 ). Sewage effluent is the major source of nutrients in the lake.
Selected samples of water eutrophication occurrence in lake, reservoir, estuary and river in the world
In China, water eutrophication occurred in 67 lakes (51.2% of the total lakes). Although the Boyanghu Lake and the Dongtinghu Lake are still mesotrophic at present, Dianchi Lake in Yunnan is possibly the most hypertropic lake in the world. In the early 1970s the water of Dianchi Lake was graded as Class III, now declined to the more inferior Class V (Lu et al., 2005 ). Taihu Lake, in China, has similar eutrophication issue. It is the third largest freshwater lake in China, located in the Yangtze River delta, one of the more developed areas of eastern China. In recent decades, because of severe pollution, water quality in Taihu Lake degraded from Class I/II in the early 1960s to Class II/III in the early 1980s and then to Class IV by the mid-1990s. At present, 83.5% of the lake area is eutrophic with an inferior Class V ranking (Liu and Qiu, 2007 ). The increasingly wider occurrence of excessive algae growth also begins earlier and lasts longer each year in Taihu Lake, and in the summer of 2007 an outbreak of blue algal bloom caused many drinking water treatment plants shut down and created a severe “water crisis event” in Wuxi City. Chaohu Lake is the fifth largest lake in China, located in central Anhui Province, and has a population of 2.3 million and more than 3000 factories in its basin. Since the 1990s, massive and rapid nutrient loading has made it one of the most eutrophic freshwater lakes in China. Jin et al.( 2005 ) reported that eutrophic trend of Taihu Lake, Chaohu Lake and Xuanwu Lake in the region of the middle and lower valleys of Yangtze River was predicated using the ecological stress model. Provided the pollution water treatment rate is 60% in 2030, approximately 30 billion ton of polluted water would still be discharged directly into the lakes. Therefore, by 2030, all the urban lakes and most of the medium-sized lakes at the urban-rural fringe areas in China may be eutrophicated or hypertrophicated.
In the region of Yangtze River delta, 80% of the rivers have been polluted and the water quality cannot meet the standards of drinking water source. The degraded water quality mainly due to eutrophication in this region has resulted in extremely serious problems for drinking water supply. In Zhejiang Province about 36 out of 88 counties are suffering from the short supply of good drinkable water sources. In 2004, water eutrophication and algae blooming even occurred in the Qiantang River, which has the highest water flow velocity in China. High concentration of phosphorus and nitrogen is gradually causing eutrophication.
Water eutrophication in rivers occurs worldwide. During the past several decades, catastrophic losses in seagrass meadows have occurred worldwide, especially in flushed estuaries, coastal embayments and lagoons where nutrient loads are both large and frequent (Burkholder et al., 2007 ; Ralph et al., 2006 ). Coastal marine ecosystems of Northern Europe are under pressure from global change (e.g., nutrient enrichment), which threatens these resources (Gowen and Stewart, 2005 ). There are many statutory obligations and strong political pressures for greatly increased emphasis on the control of nutrients levels in UK rivers because of serious problem of water eutrophication (Mainstone and Parr, 2002 ). Within Europe, many national and international initiatives have been implemented in order to reduce the inputs and effects of nutrients in waters, e.g., the European Union’s Water Framework Directive (Andersen et al., 2004 ).
Harmfulness of water eutrophication
Generally speaking, the main harmfulness of water eutrophication is that it can break out the intrinsic equilibrium of the aquatic ecosystem and lead to the damage of the water ecosystem and the gradual degeneration of its functions. As a result, it can affect water quality and make transparency of water become worse than ever. Thus, little sunlight can penetrate water body and photosynthesis of plants under the water will be weakened or even stopped. Water eutrophication can also cause the supersaturation or lack of dissolved oxygen in water, which will be dangerous to aquatic animals and cause great death to them. Eutrophic systems tend to accumulate large amounts of organic carbon causing a shift in organic matter biochemical composition (Dell′Anno et al., 2002 ). Meanwhile, because of water eutrophication, a mass of algae, mainly Cyanophyta and green algae, bloom and form a thick layer of “green scum” on water surface. Algae can release toxins and render the organic matters in water to be decomposed into harmful gases, which will poison the fish and seashell.
The harmfulness of eutrophication also includes causing the shortage supply of drinking water source by degrading water quality. When the blooming algae die, they can produce lots of algae’s toxin which is harmful to human health. Cyanobacteria toxins (cyanotoxins) including cytotoxins and biotoxins are responsible for acute lethal, acute, chronic and sub-chronic poisonings of wild/domestic animals and humans. The biotoxins include the neurotoxins; anatoxin-a, anatoxin-a(s) and saxitoxins plus the hepatotoxins; microcystins, nodularins and cylindrospermopsins (Carmichael, 2001 ). Recent investigation showed that the algae produced toxins, which are the metabolized production of Cyanotoxins, were detected in the Yangtze River, as well as many reservoirs and lakes of Yellow River valleys, apart from Dianchi Lake, Taihu Lake and Chaohu Lakes (Yu and Len, 2004 ). Besides, increased nitrite concentration in the eutrophic water will be dangerous to human health, too, as products of nitrite nitrification process is a strong carcinogen. Thus, the exacerbation of water eutrophication with the increased severity of algae blooming in surface water system has attracted great attention of both public and private sections.
FACTORS INFLUENCING WATER EUTROPHICATION
Water eutrophication is mainly caused by excessive loading of nutrients into water bodies like N and P. Excessive nutrients come from both point pollution such as waste water from industry and municipal sewage, and non-point pollution like irrigation water, surface run water containing fertilizer from farmland, etc. Increased nutrient load to water body is now recognized as a major threat to the structure and functions of near shore coastal ecosystems, and severe eutrophication problems associated with harmful algal bloom is a major manifestation. Although related to nutrient enrichment in general, the basic cause of water eutrophication is more connected to an imbalance in the load of nitrogen and phosphorus with respect to silica (Dauvin et al., 2007 ). At present, excessive TN and TP in water are considered as the only factors inducing water eutrophication, but nutrient enrichment is only the necessary but not the sufficient condition for algal boom. Eutrophication is not likely to occur if both TN and TP in water are low, but eutrophication may not occur in water high in TN and TP if other conditions such as temperature and current speed are not favorable. The influencing factors of water eutrophication include: (1) excessive TN and TP, (2) slow current velocity, (3) adequate temperature and favorable other environmental factors, and (4) microbial activity and biodiversity (Li and Liao, 2002 ). Water eutrophication may occur rapidly when all of these conditions are favorable.
Nutrient enrichment
There is clear evidence that nutrient loading to lakes, estuaries and coastal oceans has greatly increased through human activities over the past few decades and that this has caused or enhanced many of the symptoms of the aquatic ecosystem transformation known as eutrophication (Bishop et al., 2006 ). There are different opinions on the relationship of nutrient enrichment to water eutrophication and algal bloom: (1) When P concentration in water is low, it may be the limiting factor for inducing water eutrophication and algal bloom; (2) When P concentration in water increases rapidly, other may become a new limiting factor, such as pH, water depth, temperature, light, wave, wind or other biological factors; (3) The influence of N and P still lasts for a longer time because of the high development level of our society (Zhao, 2004 ).
N and P input and enrichment in water are the most primary factors to induce water eutrophication. The “experienced molecular formula” of alga is as “C 106 H 263 O 110 N 16 P” based on the chemical components of algae. N and P are the two elements which account for least proportion in the molecular formula of algae, especially P, it is the main limiting factor to control the growth of alga in water (Mainstone and Parr, 2002 ). It was reported that 80% lake and reservoir eutrophication is restricted by phosphorus, about 10% lake and reservoir eutrophication is relative to nitrogen, and the rest 10% lake and reservoir eutrophication is relative to other factors (Zhao, 2004 ). In many ecosystems, phytoplankton biomass is correlated with the availability of N or P (Cloern 2001 ; Bledsoe et al., 2004 ). The composition of phytoplankton species is also affected by the concentrations of N and P (Reynolds, 2006 ). The ratio of N:P in the water body (referred to as the “Redfield ratio”) is an important indicator of which nutrient is limiting eutrophication. If the Redfield ratio is 16:1, P is most likely the limiting factor for algal growth; lower ratios indicate that N is of great importance (Redfield et al., 1963 ; Hodgkiss and Lu, 2004 ). P has been shown to be the principal limiting nutrient for primary production of phytoplankton in many freshwater environments (Phlips, 2002 ), while N is commonly limiting in marine ecosystems (Cloern, 2001 ). However, there are many exceptions to this general pattern. In some freshwater environments, particularly in the tropics and subtropics, N has been found to be the primary limiting nutrient for phytoplankton production, due in large part to excessive P load and long growing seasons. For instances, in the Ten Mile Creek of Indian River Lagoon, where TP is >0.2 mg/L, chlorophyll a and turbidity sharply increased with addition of available N (0.2~6.0 mg/L), but not affected by addition of reactive P (Lin et al., 2008 ). The results indicate that available N is the limiting nutrient for the growth of phytoplankton at water bodies with high P. In phosphate-deficient water bodies or those having reasonably good growth of blue-green algae, which fix enough of the atmospheric nitrogen, phosphorus becomes the limiting element, because a portion of P is used to counterbalance high nitrate content (Reynolds, 2006 ). Such circumstances can be seen that no paroxysmal algal boom may break out in heavily eutrophicated water bodies with both high N and P. Thus, it is the key point to control the concentrations of both N and P reasonably for solving the problem of water eutrophication.
The variations in the chemical composition of natural waters are believed to be an important factor in regulating the abundance, composition and geographical and periodic distribution of phytoplankton. It has been considered that the growth of phytoplankton is influenced by dissolved silicate-Si (DSi) concentration in water and its ratio to nitrate. When the DSi:nitrate-N atomic ratio is near 1:1, aquatic food webs leading from diatoms which require silicate to fish may be compromised and the frequency or size of harmful or noxious algal blooms may increase. Used together, the DSi:nitrate-N ratio and nitrate-N concentration are the robust comparative indicators of eutrophication in large rivers (Turner et al., 2003 ).
Hydrodynamics
There is no relationship between water disturbance and diatom alga occurrence or its scale, but water disturbing can influence the growth of Pyrrophyta alga because Pyrrophyta alga blooms when it is grown in relatively stable water. Cai et al.( 2007 ) found that when there is no water to dilute, disturbing water itself can influence the process of eutrophication and species succession, which, however, is not related to disturbing water itself but is influenced indirectly by changing light and nutrient status. In shallow water, increased frequency of disturbance could increased the P release from the sediment, especially at high temperature (Cai et al., 2007 ). This is an instructional point to maintain beneficial alga in water. Also, tide not only can urge alga assembling but can also influence the multiplication of alga bloom through changing the concentration of nutrition in water. Zhu et al.( 2007 ) studied the effects of hydrodynamics on phosphorus concentrations in water of Taihu Lake, a large, shallow and eutrophic lake of China. They found that hydrodynamical disturbance had no significant relationship with water quality at the top layer when significant wave height was smaller than 30 cm, but it significantly increased suspended solids (SS) concentration of the bottom water layer. Concentrations of nutrients showed no positive correlation with SS concentration in the water body. Intensive sediment resuspension may not have occurred when the hydrodynamic stress on sediment was only a little higher than the critical stress for sediment resuspension. A new method for confirming the critical stress for intensive sediment resuspension and nutrient release still needs to be developed. Le Pape and Menesguen ( 1997 ) studied hydrodynamic prevention of eutrophication in the Brest Bay (France). The Brest Bay is a semi-enclosed coastal ecosystem where primary production is nutrient-limited, even if huge nutrients loading from tributaries are present. The most striking feature of the bay is the semi-diurnal tidal influence, resulting in large water exchange with the continental shelf. A historical study of the available data has shown the steadiness of this ecosystem during the last two decades inspite of increasing eutrophic conditions.
Environmental factors
A range of factors are related to water eutrophication, but the mechanisms of their influencing algal bloom are not fully understood. In many moderately eutrophicated water bodies, algal bloom occurs in some seasons or some years, when the environmental conditions are favorable. The algal bloom caused by phosphorus inputs also modifies several abiotic factors of the water body. These factors directly govern the growth, diversity and density of the biotic components. The impact of algal bloom on any one or some of these factors indirectly influences the structure and characteristics of the water bodies. The influence of nutrient inputs on some of these factors is discussed as follows:
1. Temperature and salinity are the two important factors to induce alga bloom. Alga bloom always occurs at temperature between 23 °C and 28 °C, salinity between 23% and 28%. The variation of temperature and salinity also affect algal bloom, and an important condition for algal bloom is that temperature increases and salinity decreases faster than ever in short time. From the conception of ecology, exquisite change of temperature may cause the subrogation of biological communities, thus leading to algal bloom when other environment conditions are adequate (Wang et al., 1996 ). Statistical analysis shows that the influence of temperature on algal growth rate is the largest, followed by salinity and their interaction. The process of sporangium pullulating is hypersensitive to temperature. When under adequate temperature, it can bourgeon largely and alga bloom will form very fast. Change of salinity is also influenced by the concentration of nutrition. Research shows that salinity is negatively related with NO 3 − -N, and PO 4 3− -P, but positively related with NH 4 + -N, and however, it is not very related with NO 2 − -N. In addition, average temperature in winter is highly relative with the beginning growth time of Gymnodinium, but whether it has universality to all algae still needs to be studied. In the Vistula Lagoon, salinity gradient was determined as an important factor (along with water temperature and predation by young herring) that defined the dynamics of zooplankton abundance and biomass in this estuary (Telesh, 2004 ).
2. Carbon dioxide level is one of major factors controlling water eutrophication. Cyanophytes are more capable of utilizing low levels of carbon dioxide and become more buoyant at low levels of carbon dioxide and high pH. It keeps them in the upper layers of the water column with abundant sunlight. In addition, some species produce dense mats of vegetation, inhibit the growth of other phytoplankton, and also limit the swimming of zooplankton. These factors together mean that a slow-moving freshwater ecosystem can rapidly become dominated by blue-green algae, displacing not only members of the phytoplankton but some of the animal community as well. The reduction of light reaching the lake floor also inhibits submerged and rooted macrophytes, and sediments become anoxic as large amounts of planktonic biomass are added to them (Kant and Raina, 1990 ). The fluctuations in free carbon dioxide values correspond directly with the fluctuation in the standing crop of phytoplankton. As the diversity and density of phytoplanktons increase through various months, the amount of free carbon dioxide for photosynthetic activity becomes limiting. The pH changes in these ponds are governed by the amount of free carbon dioxide, carbon trioxide, and bicarbonate (Kant and Raina, 1990 ). Inflow nutrient concentration, inflow volume and inflow water temperature show very regular and reasonable impacts on the quality of lake water (Imteaz et al., 2003 ). Yin ( 2002 ) reported that monsoons served as a flushing mechanism in two ways: (1) They reduced seasonal eutrophication by nutrient enrichment in summer, and (2) they prevented long-term (annual) accumulation of organic matter in the sediments due to nutrient enrichment in the region. Because of the monsoon-influenced processes and low phosphorus in the Pearl River estuary, the estuary and adjacent coastal waters of Hong Kong appeared to be more resilient to enrichment of nitrogen.
3. Light plays an important role in the growth, diversity and density of aquatic flora. Algal growth has been reported to increase with light intensity, and luminescence of 4000 lux was found most favorable (Shen, 2002 ). As eutrophication progresses, a decline of submerged macrophytes occurs in many shallow water bodies, probably due to low light intensity caused by algal blooming. It is suggested that the adaptation strategy of Potamogeton maackianus under a certain range of low light stress is to accelerate the elongation of the main and lateral shoots and to increase their density (Ni et al., 1999 ). The light has been almost completely absorbed by the plankton of the top few meters, so that too little light penetrates to the thermocline and beyond to support photosynthesis. However, there is a rain of corpses into the deep water, whose decomposition requires oxygen. Since the deep water is cut off from the air until fall overturn, an oxygen deficit develops in the deep water, and the bottom mud is reduced. Eutrophication in an estuary is a complex process, and climate change is likely to affect each estuary differently due to interactions with nutrient loading and physical circulation. Hence, it is essential to consider the effects of climate change on the context of individual estuarine function to successfully manage eutrophication (Howarth et al., 2000 ).
There are other factors like pH and dissolved oxygen affecting water eutrophication (Khan and Ansari, 2005 ). The minima and maxima in the concentration of dissolved oxygen are found to be directly related to the maxima and minima of the phytoplankton, The direct relationship between phytoplankton and dissolved oxygen content has been observed by a number of researchers (Khan and Ansari, 2005 ). pH is a plant growth limiting factor. The change in pH is directly related to the availability and absorption of nutrients from solution. Ionization of electrolytes or the valence numbers of different ion species are influenced by changes in pH. An acidic pH has been reported to promote growth of Spirodelapolyrrhiza at a faster rate, but high pH values promote the growth of phytoplankton and result in bloom. It must be pointed out that many factors influencing eutrophication are relative and affect each other.
Microbial and biodiversity
Microbial activity is the inducement factor to alga bloom (Paerl, 1998 ; Paerl et al., 2003 ). It can enhance abundant breeding of alga bloom. Nutrient-enhanced microbial production of organic matter, or eutrophication, is frequently accompanied by altered microbial community structure and function (Paerl, 1998 ). The amount of microbial biomass is positively related to the content of organic matter and the amount of plankton in eutrophicated water. There exists certain intrinsic relationship between the amount of bacteria and the occurrence of eutrophication. The decomposition of organic matter by bacteria activities can produce nutrients and organic substances, which may promote algal bloom breaking out. Of course, it may also produce some toxic substances, which are harmful to other algal species, so that it will selectively enhance the bloom of some algae to become preponderant species and subsequently eutrophication will occur. It may be relative with the decomposing of bacteria biomass, which can promote effective circulation of nutrients when alga bloom and eutrophication occur under lower concentrations of nutrients. Chang et al.( 2005 ) demonstrated that impose of submerged macrophyte in combination of immobilized nitrogen cycling bacteria could effectively reduce chlorophyll a concentration and increase water transparency. Marshland drainage channels (=ditches) in the UK are relicts of a once extensive habitat whose management requires quantitative information on the ecology of marshland organisms. Distribution of these organisms in wetlands worldwide can reflect natural water quality, vegetation and anthropogenic factors (Watson and Ormerod, 2004 ). Acrophyte-specific richness and abundance increased along an upstream-to-downstream zonation, which was characterized by an increase in mineralization and nutrient level (Thiébaut and Muller, 1998 ). In hyper-eutrophicated water body, remarkable improvement in water quality and inhibition on algal growth was obtained by introducing nutrient cycling bacteria in proper combination with floating hydrophyte (Chang et al., 2006 ).
A comparison of aquatic macrophyte diversity of two streams reflected the impact of human-induced perturbations (fish farms, domestic sewage) in such weakly mineralized and poorly buffered waters. Disturbed sites with very high nutrient loading were characterized by low vascular plant richness and by the absence of filamentous algae (Thiébaut and Muller, 1998 ). Vădineanu et al.( 1992 ) studied the phytoplankton and submerged macrophytes in the aquatic ecosystems of the Danube Delta and found that the species changes were linked to accelerated eutrophication of the lakes, with increased phosphorus loading and a reduction in the N/P ratio. Distinct changes were observed in the macrophyte species composition in response to phosphorus enrichment (Vaithiyanathan and Richardson, 1999 ). Marshes in the unenriched and enriched areas were dominated by Ladiumjamaicense and Typha domingensis , respectively. Open-water areas were characterized by Eleocharis spp., Utricularia spp., Chara zeylanica and Nymphaea odorata in ligotrophic areas and by floating plants and Polygonum spp. in eutrophic areas. A shift in primary producers from eelgrass to macroalgae in response to increased nutrient loading altered the habitat, physicochemical structure and food webs. The nitrogen decreased shoot density and biomass of the eelgrass and promoted a record increase in the algal biomass (Deegan et al., 2002 ). Enhanced nutrient concentrations and loading have been observed in several coastal areas of the North Sea, resulting in increased production and changes in the species composition of phytoplankton (Colijn et al., 2002 ). Garg et al.( 2002 ) studied aquatic flora in three lakes of Bhopal (Upper Lake, Lower Lake and Mansarovar Lake) in India and assessed the potential fertility of the lentic water and its aquatic flora. Eutrophication was highest in Mansarovar Lake. The observations of Garg et al.( 2002 ) indicated that different species of phytoplankton could subsist up to a certain nutrient level, beyond which competition between cyanophytes and other algae enhanced and eliminated the sensitive plankton flora.
RESEARCH PERSPECTIVES
The problem of water eutrophication has become more and more severe worldwide, but the mechanism of its occurrence has not been fully understood. The limited knowledge of water eutrophication processes will add difficulties for the prevention and remediation of water eutrophication. Therefore, more researches should be turned to the mechanisms of water eutrophication under different watershed conditions. For example, the mechanisms of the adsorption and release of the contaminants in sediments should be clarified, which named inner pollution converging in water bodies, especially the absorption and release of P in sediments; the mechanism of the excessive production of algae and Cyanobacteria, especially excessive production of blue-green algae in water should be further studied, which is the key for the prevention of algae and Cyanobacteria growth. Also, the guidelines for estimating eutrophication are still very incomplete. Comprehensive guidelines for assessing eutrophication should be established by considering various factors in combination with the development of economy and society, especially in modern society ecology and health are paid more and more attention in order to avoid adverse influence on the sustainable ecological development and human health to the best of our abilities. In view of the high level of nutrients already polluted into lakes, reservoirs, estuaries, etc., understanding the functions of the factors influencing algal growth and bloom will certainly help controlling algal bloom even at high nutrient burden in surface water bodies.
* Project supported by the Key Project from the Ministry of Education of China (No. 705824), the Project from Science and Technology Bureau of Zhejiang Province (No. 2006C13059), and a grant from the St. Lucie River Water Initiative (SFWMD contract No. OT060162), USA, in part
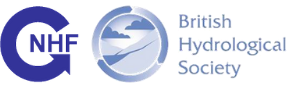
- Previous Article
- Next Article
INTRODUCTION
Study area and data, methodology, results and discussion, conclusions, acknowledgements, effects of rainfall-runoff pollution on eutrophication in coastal zone: a case study in shenzhen bay, southern china.
- Article contents
- Figures & tables
- Supplementary Data
- Open the PDF for in another window
- Guest Access
- Cite Icon Cite
- Permissions
- Search Site
Hongliang Xu , Ying Zhang , Xiuzhen Zhu , Mingfeng Zheng; Effects of rainfall-runoff pollution on eutrophication in coastal zone: a case study in Shenzhen Bay, southern China. Hydrology Research 1 August 2019; 50 (4): 1062–1075. doi: https://doi.org/10.2166/nh.2019.012
Download citation file:
- Ris (Zotero)
- Reference Manager
The concentration of human activities in coastal cities results in the increase of nutrient salts released into the coastal environment and is identified as a major environmental problem for coastal zone management. Large amounts of nitrogen and phosphorus are transported by rainwater-runoff from urban catchments to coastal zones during episodic rainfall events inducing eutrophication problems and increasing the risk of red tide occurrence. This study used a coupled model based on the Storm Water Management Model (SWMM) and Environment Fluid Dynamic Code (EFDC) to simulate the rainfall-runoff pollution load and its effects on eutrophication in Shenzhen Bay, southern China. A storm event of 2014 was used to build the modeling scenarios and thus analyzed the spatial-temporal variation of the rainfall-runoff pollution. The results indicated that: (i) rainfall-runoff pollution loads accounted for 60–80% of the total pollution loads, and rainfall-runoff pollution can result in a short-term impact pollution load on the receiving seawater body; (ii) the transportation of nutrient salts in the coastal zone and the nutrient salts absorbing process by algae are at different times, which suggests urban rainfall-runoff pollution has evidently an effect on variation of the concentration of chlorophyll-A in the bay, and with increasing distance to the city, the seawater body is gradually less affected by rainfall-runoff pollution.
Rapid urbanization in coastal zones can result in increased emissions of nutrients, such as phosphorus (P) and nitrogen (N), into the surrounding environment. Pollutants (mainly N and P) in urban areas can be washed away by storm runoff, flow into natural water bodies and, ultimately, cause the eutrophication of lakes, rivers, and coastal waters, and meanwhile are a threat to ecosystem security.
Urban storm runoff is the main driving force for the migration and conversion of nutrients in urban areas ( Brinkmann 1985 ). Nutrients, especially those containing N and P, exist widely in the natural environment. Moreover, industrial and agricultural activities also emit a large amount of nutrient salts into the surrounding environment (in which the nutrient salts released into the atmosphere play an important role in the formation of aerosols) and cause pollution. The nutrient salts accumulate in soil and water bodies and on the rough earth surface via pollutant emission and atmospheric dry deposition, etc., during non-rainy days. During rainfall events, aerosol particles get into cloud droplets through working as cloud nuclei, then the falling rain droplet particles collide with aerosol particles through Brownian diffusion, interception, impaction, and turbulent diffusion ( Seinfeld et al. 1998 ). Finally, the nutrient salts are discharged into the surrounding coastal environment by leaching and wash off via surface flow and sewage system.
In urban areas, the surface runoff is generated and polluted on the ground and then flows into the municipal drainage system during rainfall events, and is finally discharged into natural waterbodies. Many studies have focused on the investigation of the formation and characteristics of nutrient loads on various surfaces, such as roofs and roads, in urban areas ( Carpenter et al. 1998 ; Gnecco et al. 2005 ; Hathaway et al. 2012 ). Studies on the re-mobilization of sediments in stream and river channels have indicated that the initial scouring effect plays an important role in the formation of polluted surface runoff ( Bertrand-Krajewski et al. 1998 ). To quantify the impact of polluted urban surface runoff on waterbodies, many empirical or conceptual process models (e.g., SWMM (Storm Water Management Model) and HSPF (Hydrological Simulation Program-Fortran)) have been developed to simulate the wash-off process of accumulated pollutants, and the transport and conversion processes of the polluted runoff in municipal sewage systems and in river channels. Burian et al. (2001) investigated the suitability of integrating deterministic models to estimate the relative contributions of atmospheric dry and wet deposition onto an urban surface and the subsequent volumes removed by stormwater runoff. Bergman et al. (2002) modeled pollutant loads during storm events in the South Prong watershed, USA, using HSPF and found that the prediction error of TSS (total suspended solids) and TP (total phosphorus) was 3% and 24%, respectively. Temprano et al. (2006) studied pollution fluxes during rainy weather in a combined sewer system catchment in Santander, Spain, using SWMM, and indicated that the accuracy of the total simulated loads of SS (suspended solids), COD (chemical oxygen demand) and TKN (total Kjeldahl nitrogen) at the end of the rainfall events were 93%, 95%, and 78%, respectively. Moreover, the loads emitted during the first-flush events were determined to account for 65%, 57%, and 54% of the total polluting loads of COD, SS, and TKN, respectively.
In coastal cities, pollutants in storm runoff will eventually be transported into the natural waterbodies such as sea, rivers, and lakes, and will result in the deterioration of water quality ( Josefson & Rasmussen 2000 ; Kemp et al. 2005 ; Bricker et al. 2008 ; Chen et al. 2017 ). Storm runoff contains many kinds of pollutants (e.g., N, P, heavy metals, petroleum hydrocarbons, etc.). The increase of nutrient concentrations (mainly N and P) which are caused by the polluted surface runoff can easily lead to a red tide breakout under appropriate weather and environmental conditions ( Villarino et al. 1995 ; Conley et al. 2009 ). It should be noted that storm runoff not only conveys nutrient salts to the coastal waters, but also contains toxic pollutants (e.g., heavy metals, organo-chlorine pesticide, polycyclic aromatic hydrocarbons, etc.) that can inhibit algal growth but are low in concentrations. After the dilution by coastal waters, the toxic pollutants in urban storm runoff pose an even more subtle influence on inhibiting algal growth. Turner & Rabalais (2004) studied the annual loads of C, N, P, silicate, TSS and their yields in six watersheds of the Mississippi River Basin, USA, using water quality and water discharge records from 1973 to 1994. They found that the increase of inorganic nitrogen and bio-available phosphate resulted in algae blooms in the northern Gulf of Mexico. Bowes et al. (2005) investigated the seasonal variation of TP, PP (particulate phosphorus) and SRP (soluble reactive phosphorus) concentrations at three points along the River Swale in England, and pointed out that P from storm runoff was the dominant factor causing eutrophication in the intertidal zone of the river mouth. Maillard & Santos (2008) evaluated the effects of land use and land cover on the quality of nearby stream water in a semi-arid watershed in southeastern Brazil. The results suggested a strong relationship between land use and land cover on turbidity, along with higher concentrations of N and fecal coliforms. This demonstrated that storm runoff during the rainy season conveyed more organic material into the sea and led to the deterioration of seawater quality. Saniewska et al. (2018) investigated the impact of intense rains and flooding on the input of mercury into the coastal zone of the southern Baltic region and found that the decreased retention of mercury during intense rainfalls demonstrated mercury elution from the catchment. Meanwhile, floods, melting snow, and development of urban infrastructure and farmlands increases also have a tremendous impact on the outflow of mercury from the catchment. Rossi et al. (2018) investigated the space–time dynamics of N pollution in a Mediterranean gulf by means of δ 15 N variation and found that coastal and offshore areas were vulnerable to freshwater-transported nutrients, consistent with terrestrial hydromorphology and sea surface-water circulation.
In recent decades, many marine ecological dynamic models have been developed to simulate, predict, and manage the coastal zone environment. For example, USEPA (United States Environmental Protection Agency) recommends the use of WASP (Water Quality Analysis Simulation Program) and MIKE (developed by DHI (Dansk Hydraulisk Institut)) to model the variation of water quality in coastal zones. The models have been successfully applied to simulate the eutrophication of estuary water bodies in many areas, such as Chesapeake Bay ( Hamrick & Wu 1997 ) and the river mouth of the Neuse River ( Wool et al. 2003 ) in the USA, Kwang-Yang Bay, South Korea ( Park et al. 2005 ), Narva Bay, Estonia ( Lessin & Raudsepp 2007 ), the Perth coastal margin, south-western Australia ( Machado & Imberger 2014 ), Virginia Beach ( Johnson & Sample 2017 ), etc. With the help of these prediction models, many studies have indicated that storm runoff contributed 55% of the total pollutant loads in coastal zones in the USA and investigations in Europe have also provided similar conclusions ( Goonetilleke et al. 2005 ).
Based on the foregoing studies, it is evident that many investigations took into account storm runoff pollution in coastal urban areas using various methods. The formation mechanisms of storm runoff pollution and their characteristics have been studied extensively. Meanwhile, many computer models based on hydrological, physical, and chemical processes are being developed to analyze the spatial-temporal variation of pollutant outputs at basin scale. Furthermore, studies on eutrophication in coastal zones have mainly focused on the variation of the flux of nutrients, their transport and conversion mechanisms, and the relationship between algal growth and environmental factors. However, the effect of nutrients (mainly N and P) carried by the storm-generated surface runoff on the variation of eutrophication (reflected by the concentration of chlorophyll-A) in coastal zones has not yet been the subject of much research attention, and the spatial and temporal variation of algae proliferation after a single storm event in offshore areas remains unclear. The above considerations form the basis of this study, and the objectives of this study were to: (1) study the spatial-temporal variation of nutrient salts in an offshore area under storm runoff pollution; and (2) assess the use of coupled simulation and prediction models in chain on analyzing the variation of algae blooms that respond to the nutrient salts from urban storm runoff to coastal recipient.
The Shenzhen Bay Basin is located at 22.36°–22.61°N and 113.87°–114.18°E, in the eastern Pearl River Delta, southern China ( Figure 1 ). Shenzhen Bay is a semi-closed gulf, and the basin covers a 607 km 2 land area in Shenzhen and Hong Kong, while the bay itself is a 15 km long and 5.5 km wide semi-closed bay of 83 km 2 and mean depth of 3 m. Mountains and hills are distributed along the north of the basin and are the sources of the rivers flowing into the bay. The basin is dominated by a subtropical maritime monsoon climate with an annual mean temperature of 22.4 °C and a multi-year mean precipitation of 1,883 mm. The rainy season is from April to September and accounts for approximately 80% of the annual rainfall. The terrain in the basin results in floods that have a short confluence time and a high peak runoff volume during rainy season storms. The basin is highly urbanized with a population of nearly 3.5 million. Due to the lack of effective regulation, a large amount of wastewater from industrial activities without treatment pollutes the rivers and leads to a large amount of terrigenous pollutants (including nutrients, petroleum hydrocarbon, heavy metals, etc.) being emitted into the bay.

Study area: Shenzhen Bay Basin.
Nutrient salts and chlorophyll-A concentrations, which were monitored online in 2014 from eight buoys in Shenzhen Bay ( Figure 1 ), were used to analyze the variation of the seawater quality in the bay.
The surface runoff pollution data were monitored on April 5th 2012 and April 16th 2014, respectively. The concentrations of nitrate (NO 3 -N), ammonia nitrogen (NH 3 -N), and phosphate (PO 4 3− ) in the surface runoff originating from typical industrial land, residential land, and roads were tested under different periods of the storm events.
The land-use map includes five kinds of land-use types: industrial land, residential land, road, water body, and green landscapes. It was derived from Landsat TM images by using supervised classification method in ENVI, 90 m resolution digital elevation model (DEM) of Shenzhen Bay Basin, municipal sewage system map, weather data, monitored surface runoff pollution data, point-source pollution data, river water quality data, seawater quality data, tide level, and seabed elevation, which were used as input of SWMM and EFDC (Environment Fluid Dynamic Code), and modeled the variation of pollutant loads in the rivers and in the bay during storm events.
Environmental models
As the migration and conversion of the nutrient salts in storm runoff relate to both the land and sea areas, significant interactions between sea and land can be observed. To analyze the effects of storm runoff pollution in coastal urban areas on the coastal water environment, this study made use of two models, SWMM and EFDC, to simulate storm runoff pollution and eutrophication in Shenzhen Bay, respectively.
USEPA's SWMM is a widely used computer model that accounts for various hydrologic processes that produce runoff from urban areas and estimates the production of pollutant loads associated with stormwater runoff ( Chang et al. 2015 ; Guan et al. 2015 ; Shuster et al. 2015 ; Estalaki et al. 2016 ). It is a dynamic hydrologic–hydraulic water quality simulation model that can be used for single events or for long-term (continuous) simulation of runoff quantity and quality from primarily urban areas. The runoff component operates on a collection of sub-catchment areas that receive precipitation, and generate runoff and pollutant loads. The routing portion transports this runoff through a system of pipes, channels, storage/treatment facilities, pumps, and flow regulators. The quantity and quality of runoff modeled in SWMM is generated within each sub-catchment. The model tracks the flow rate, flow depth, and quality of water in each pipe, and then channels it during a simulation period made up of multiple time steps.
EFDC is a state-of-the-art multi-functional surface water modeling system developed by USEPA that can be used to simulate aquatic and coastal systems in one, two, and three dimensions. The model includes hydrodynamic, sediment-contaminant, and eutrophication components. It has been successfully applied in the modeling of environmental changes in water bodies (e.g., rivers, lakes, reservoirs, wetlands, estuaries, and coastal ocean regions) to support environmental assessment and management ( Liu et al. 2008 ; O'Donncha et al. 2013 ; Chen et al. 2016 ). The computational scheme of the model utilizes an external–internal mode splitting, used to solve horizontal momentum equations and the continuity equation on a staggered grid. The transport equations for turbulence intensity, turbulence length scale, salinity, temperature, suspended sediments, dissolved and adsorbed contaminants, and dye tracer are also solved, using a fractional step scheme with implicit vertical diffusion and explicit advection and horizontal diffusion ( EFDC User Manual 2007 ).
Process of modeling eutrophication
This study made use of the output of SWMM as the input of EFDC to model the impact of storm runoff pollution on the coastal zone. First, the study area was divided into two sub-systems, i.e., the urban area and the offshore area. The polluted storm runoff was produced in the urban area, and the offshore area received the polluted runoff and provided the growth environment of algae. The collected data (regarding the river network, municipal sewage system, point source pollution, rainfall, tide level, salinity, water quality, solar radiation, etc.) were analyzed and the key interaction processes that effect eutrophication between the urban and offshore area were identified. Finally, the SWMM and EFDC models were calibrated and then applied in various scenarios to analyze eutrophication in Shenzhen Bay during and after storm events.
Calibration and validation of SWMM and EFDC
SWMM was calibrated based on monitored storm runoff pollution data and meteorological data. Meanwhile, the model used four parameters ( Table 1 ) to simulate the water quality indices (NO 3 -N, NH 3 -N, and PO 4 3− ) in three different land surfaces (i.e., road, industrial land, and residential land).
The parameter values calibrated in SWMM
a c 1 is maximum cumulant; c 2 is subsaturation cumulative time; c 3 is coefficient of scouring; c 4 is wash off index.
b 0.32/0.22/0.08 represents the parameter values in modeling NH 3 -N/NO 3 -N/PO 4 3− .
The validated NSE (Nash–Sutcliffe model efficiency coefficient) values were 0.65–0.88, indicating that the SWMM was suitable for the simulation of storm runoff pollution in the urban area in this study. Figure 2 shows an example of the variation of the validated pollution load under a storm event on three kinds of pavements (the black lines represent the simulated values while the hollow dots represent the concentration values of the nutrient salts in water samples collected from the curbside storm drains during storm events). It can be seen that SWMM can satisfactorily model the nutrient salts accumulated on the industrial land and the residential land in the urban area in the basin, while the simulation of the accumulated NH 3 -N and PO 4 3− on the road produces relatively large errors, especially the monitored values of PO 4 3− concentration appear to be dispersed in the figure and not concentrated along the modeled curve ( Figure 2(a2) and 2(a3) ). It should be noted that different land surfaces host different human activities which result in different pollutant accumulation, e.g., high values of NO 3 -N concentration (maximum 5.26 mg/L) was monitored on road surfaces due to a large amount of automobile exhaust emitted towards the road ( Figure 2(a1) ); high values of NO 3 -N and NH 3 -N concentration (maximum 5.04 mg/L and 5.06 mg/L, respectively) were monitored due to the sampling sites of industrial land located in a chemical industry park ( Figure 2(b1) and 2(b2) ); The monitoring sites of residential land were located near several restaurants which occupied parts of the sidewalk to run their business. Therefore, the pavement was polluted by the kitchen waste and higher values of NH 3 -N and PO 4 3− concentration (maximum 9.67 mg/L and 0.43 mg/L, respectively) were detected in residential land ( Figure 2(c2) and 2(c3) ).

The simulated nutrient salts on roads, industrial land, and residential land in SWMM.
However, for the study area located in the highly industrialized Pearl River Delta, sulfate (S), NO 3 -N, and NH 3 -N are the major water-soluble ions in particulate matter in the atmosphere ( Dai et al. 2013 ). The particulate matter accumulates on the pavements via dry deposition, resulting in the concentration values of NO 3 -N and NH 3 -N in storm runoff from different land use not to appear obviously different.
Hydrodynamics and salinity play important roles in affecting the migration and diffusion of pollutants from urban storm runoff into estuaries. Accurate simulation of the hydrodynamic conditions and salinity is the basis of modeling the eutrophication in the bay. Monitored seawater quality data from 6th–15th in June and 16th–23rd in April, 2014 were used to calibrate and validate the EFDC model, respectively. In addition, 15 parameters were calibrated ( Table 2 ) in the model after sensitive analysis to simulate the variation of the water quality in the bay.
The parameter values calibrated in EFDC
Table 3 shows the statistical indices during EFDC's validation period. It can be seen that EFDC presented the smallest relative error ( RE ) ( RE = 0.02) when modeling PO 4 3− , while the largest deviation appears when modeling salinity ( RE = 2.24). The NSE values are all larger than 0.6, which indicates that the EFDC can satisfactorily reflect the dynamic variation of the modeled seawater quality data as compared to the monitored data.
The validation results of the simulated hydrodynamic and seawater quality indices in Shenzhen Bay in EFDC
Variation of the water quality in Shenzhen Bay before and after rainfall
The monitored nutrient salts data during the wet and dry seasons of 2014 ( Figure 3 ) in Shenzhen Bay indicated that the nutrient salts' concentrations during the wet season were noticeably higher than those during the dry season. This was attributed to the fact that the sediments in the drainage pipes and river channels flowed into the bay, and together with the poor hydrodynamic conditions in the bay, resulted in the deterioration of the seawater quality. It can be seen that the concentrations of NO 3 -N, inorganic nitrogen, and PO 4 3− were 0.51–1.83 mg/L, 0.78–2.23 mg/L, and 0.09–0.19 mg/L during the wet season, respectively, while they were 0.07–1.42 mg/L, 0.07–2.09 mg/L, and 0.03–0.17 mg/L during the dry season, respectively.

The monitored nutrient salts in the wet season and dry season in 2014 in Shenzhen Bay.
More specifically, the monitored inorganic nitrogen and PO 4 3− concentrations before and after four rain events in one buoy (point B in Figure 1 ) are presented in Table 4 . It can be observed from the table that the rain volume, rain duration, and the duration of the antecedent dry period clearly impact the concentrations of N and P in the offshore seawater. The longer the antecedent dry period was, the larger were the pollutant loads accumulated on the urban ground surface that can then be washed off and transported into the sea. The pollutants were accumulated on rough surfaces and also concealed in the crevices of pavements, therefore, only a proportion of the cumulative pollutants can be washed off by surface flow; the higher the rain volume and the longer the rain duration were, the larger were the accumulated pollutant loads to be washed off. However, the relationships between the concentration of pollutants and the rain event characteristics are complex, and many factors (e.g., weather conditions and hydraulic conditions) may significantly affect the seawater quality after the pollutants have migrated into the sea.
The monitored inorganic nitrogen and PO 4 3− concentration before and after rain events in one buoy in Shenzhen Bay
Impacts of storm events on the eutrophication of Shenzhen Bay
Outputs of pollutant loads during storm events.
A typical storm event of 72 mm accumulated rainfall with its antecedent dry period being longer than 5 days (occurring on March 30th, 2014, 2 hour duration and return period of one year) was selected to model the impact of storm runoff on the eutrophication in Shenzhen Bay. The simulation results suggested that the whole urban area in the basin output in total 14.54 tons of NH 3 -N, 8.93 tons of NO 3 -N, and 2.05 tons of PO 4 3− , respectively, into Shenzhen Bay due to the storm of March 30, 2014. In addition, we modeled the monthly output load of nutrient salts in storm runoff in 2014 by using SWMM ( Figure 4 ). It is seen from Figure 4 that the rainfall in 2014 was mostly concentrated in the March to September period, and accompanied by the storms, the basin output most of the nutrient salts load during this period in the year. Most of the nutrient salts load was eventually transported into the bay and caused the deterioration of the bay water quality. From March to September, the output load of NH 3 -N, NO 3 -N, and PO 4 3− was 1,012.5 tons, 673.8 tons, and 87.6 tons in the storm runoff, respectively, which accounted for 87% of the annual total nutrient salts load (2,039 tons). It should be noted that river sediment is another important source of nutrient salts. In addition, the polluted muddy bottom layers of the rivers contain relatively high concentrations of N and P, and these can be released into waterbodies, particularly under flood conditions.

The monthly rainfall amount and output load of nutrient salts in storm runoff in the study basin in 2014 modeled by SWMM.
Furthermore, the simulated storm events (see Appendix, Table A1 and Figure A1, available with the online version of this paper), with different return periods were used to model the pollutant loads in storm runoff in the SWMM. It can be seen in Figure 5 that the loads of NO 3 -N, PO 4 3− , and NH 3 -N evidently increased with the increase of the return periods. The loads of NH 3 -N, NO 3 -N, and PO 4 3− increased rapidly from 10.22 tons, 5.92 tons, and 1.03 tons, respectively, to 13.58 tons, 8.01 tons, and 1.86 tons, respectively, when the return periods of the storm events increased from 0.1 year to 0.5 year. Meanwhile, the loads of the pollutants exhibited a slight increase when the return period was larger than 0.5 year.

The simulated load of nutrient salts under the storm events with different return periods.
Table 5 shows the proportion of the pollutant loads modeled for the designed storm runoff as part of the total pollutant loads that migrated into the sea. Under different designed storms, the pollutant loads produced by runoff were much larger than those produced by point-sources. The pollutant loads from runoff account for approximately 60–80% of the total pollutant loads during the concentration time and thus impacted the offshore receiving seawater quality obviously.
The proportion of modeled pollutant load from designed storm runoff in the total pollutant load (%)
Impact of rainfall on the eutrophication in the bay using monitored data
The concentrations of NO 3 -N, NH 3 -N, PO 4 3− , and chlorophyll-A were monitored before and after the storm event that occurred on March 30th, 2014 in Shenzhen Bay. Figure 6 presents the distribution of the concentrations of nutrient salts and chlorophyll-A in the bay on the first day prior to the storm event (marked as −1d) and on the first, third, fifth, seventh, and ninth following days (marked as +1d, +3d, +5d, +7d, +9d). It can be seen that the concentrations of nutrient salts evidently increased in the estuary area of the Shenzhen River while they remained unchanged in other areas of the bay on the first day after the storm; with the nutrient salts gradually being diffused into the bay under the effect of water exchange between the inside and outside of the bay (and there was no rainfall during these days), their concentrations decreased in the estuary area while they increased in the middle and outer parts of the bay; and with the water mass with high concentrations of nutrient salts moving towards the outer part of the bay and gradually being diffused, the nutrient salts' concentrations decreased in the inner part of the bay while they increased in the middle and outer parts during the fifth to seventh days after the storm event. Meanwhile, the distribution of the nutrient salts' concentrations in the various parts of the bay gradually became homogeneous, and on the ninth day after the rainfall, the distribution of nutrient salts' concentrations was similar to the values measured before the rainfall.

The distribution of the monitored nutrient salts and chlorophyll-A in Shenzhen Bay after a storm event.
In addition, it is seen that the concentrations of NO 3 -N and NH 3 -N in the inner bay (especially in the estuary area of Shenzhen River) on the first day following the storm event ( Figure 6(b1) and 6(b2) ) were approximately as high as they were within the same range as the concentration values in Figure 2 . Moreover, the concentrations of PO 4 3− ( Figure 6(b3) ) were obviously higher than the concentration values monitored in the water samples collected from the three kinds of pavements ( Figure 2(a3), 2(b3) , and 2(c3) ). This was mainly due to: (1) the land-use types of the upstream and left bank of Shenzhen River being vegetation and water bodies (farmland, grassplots, and fishponds), which output large amounts of nutrient salts into the river and coastal waters in storms; and (2) the storm and flood changed the hydro-dynamic conditions of the rivers and the bay, consequently, the polluted bottom mud released large amounts of N and P into the waterbody. As well, Figure 6(b3) , to some extent, indicates that the paved surfaces were not the main source of PO 4 3− as its biogeochemical cycle has no significant atmospheric component.
In the case of the concentration of chlorophyll-A, due to the fact that a large amount of flood volume diluted the seawater near the estuary of the river, it evidently decreased during the first day after the storm event, while in other parts of the bay, the chlorophyll-A concentration remained unchanged. By the third day after the storm event, the tide and the diffusion of the nutrient salts resulted in the concentration of chlorophyll-A being gradually reversed to its normal levels in the estuary area of the bay. Due to the movement of the seawater, the nutrient salts migrated into the inner and middle parts of the bay; consequently, the chlorophyll-A concentration was raised in the inner and middle parts of the bay, while it remained unchanged in the outer part of the bay on the third day after the rainfall. On the fifth to seventh days after the rainfall, due to the accumulation of nutrient salts and the suitable weather conditions, the algae bloomed in the bay and the chlorophyll-A concentration achieved its peak value on the fifth day after the storm event. On the ninth day after the storm event, the chlorophyll-A concentration recovered to its normal levels, similar to that measured before the rainfall event.
In general, the inner part of the bay was significantly affected by the nutrient salts brought about by storm runoff soon after the storm event, and the middle part of the bay was only slightly affected by storm runoff pollutants. Meanwhile, the storm runoff pollutants had no influence on the water quality of the outer part of the bay. The variation of the distribution of chlorophyll-A concentration in Shenzhen Bay suggested an entirely different phenomenon taking place. The chlorophyll-A concentration in the middle part of the bay was evidently higher than that in the other parts of the bay, and its peak appeared 5 days after the rainfall event occurred.
Impact of rainfall on bay eutrophication, modeled by EFDC under the designed rainfall
The designed rainfall events and the output of SWMM were used in EFDC to model the variation of the nutrient salts and chlorophyll-A concentrations in the bay. The analysis took into account the peak values of nutrient salts and chlorophyll-A concentration in different parts of the bay (evaluated at points A1, A2, and A3 in Figure 1 ) before and after the simulated rainfall event (indicated as CA PB and CA PA , respectively). Point A1 is located in the estuary, point A2 in the middle part of the bay, and point A3 in the mouth of the bay. The time between the start of the rainfall event and the moment when the peak values of chlorophyll-A concentration accrued is indicated as T1 and the time between the peak values of chlorophyll-A concentration and its recovery to normal levels as T2.
Tables 6 – 9 show the variation of CA PB , CA PA , T1, and T2, as modeled by EFDC under the designed storm events with different return periods. The simulation results indicate that the nutrient salts' concentrations in the estuary area (represented by point A1, Figure 1 ) increase rapidly and achieve peak values in about 6 hours after rainfall. The peak nutrient salts' concentrations increase with the increase of rain volume, where the concentrations of NH 3 -N, NO 3 -N, and PO 4 3− increase from 3.31, 3.45, and 0.69 mg/L to 4.25, 4.32, and 1.06 mg/L, respectively. In approximately 4 days (89–99 hours) after the peak nutrient salts' concentration values appeared, the concentrations returned to their normal levels, similar to the peak values before rainfall. This phenomenon indicates that the storm runoff pollution continuously influences the nutrient salts' concentrations for 4–5 days.
The variation of NH 3 -N concentration before and after designed storm events in Shenzhen Bay
a 3.01/1.12/0.59 represents the concentration in estuary area/middle part/outer part of the bay.
The variation of NO 3 -N concentration before and after designed storm events in Shenzhen Bay
The variation of PO 4 3− concentration before and after designed storm events in Shenzhen Bay
The variation of chlorophyll-A concentration before and after designed storm events in Shenzhen Bay
In the middle part of the bay (represented by point A2, Figure 1 ), the nutrient salts' concentrations gradually increased and achieved peak values within about 3 days (68–72 hours) after the rainfall. The concentrations of NH 3 -N, NO 3 -N, and PO 4 3− increased from 1.2, 1.26, and 0.16 mg/L to 1.62, 1.73, and 0.21 mg/L, respectively. In approximately 3 days (69–74 hours) after the peak nutrient salts' concentration values appeared, the nutrient salts' concentrations returned to their normal levels, similar to the values before rainfall. However, the simulation results indicated that the storm runoff pollution essentially has no impact on the nutrient salts' concentrations in the outer part of Shenzhen Bay (represented by point A3, Figure 1 ).
In the case of the modeled chlorophyll-A, the concentration in the estuary area continuously decreased and reached its lowest values in about 8 hours due to the flood continuously flowing into the river mouth, diffusing the concentration of chlorophyll-A. Afterwards, the concentration of chlorophyll-A returned to its normal levels (similar to the concentration before rainfall) in about 1 day after the lowest value appeared. In the middle part of the bay, the chlorophyll-A concentration remained unchanged during about 3 days after the rainfall, subsequently increased on the fourth day after the rainfall and achieved its peak on the fifth day (114–123 hours) after the rainfall event. Meanwhile, the peak concentration values increased from 20.9 mg/L to 28.2 mg/L with the increase of the volume of rain in comparison with the peak concentration of 10.2 mg/L before rainfall. Nonetheless, the chlorophyll-A concentration in the outer part of the bay remained essentially the same.
In general, the modeled results from EFDC indicated that the storm runoff pollution influenced the water quality in the inner and middle parts of the bay obviously, but had essentially no impact on the water quality in the outer part of the bay. Furthermore, the variation of the nutrient salts' concentrations directly affected the variation of the chlorophyll-A concentration.
This study investigated the impacts of storm runoff pollution on eutrophication in Shenzhen Bay using monitored data and environmental models. The variation of the seawater quality was sensitive to the pollutants migrating as a result of the storm runoff. The following can be concluded:
EFDC driven by the output of SWMM (i.e., the pollutant loads in storm runoff) can satisfactorily model the variation of nutrient salts and chlorophyll-A concentrations in Shenzhen Bay.
Under the simulated storm events with the return periods varying from 0.5 to 2 years, the pollutant loads (NO 3 -N, NH 3 -N, and PO 4 3− ) in storm runoff accounted for approximately 60–80% of the total pollutant loads in the urban area of the basin, and were significantly higher than the pollution produced by point-sources.
After the pollutant load migrated into Shenzhen Bay, the time lag between the pollutant loads generated from runoff and the algal bloom can be observed: the diffusion of pollutants by hydrodynamic forces took a certain period of time and the nutrient salts absorbed by algae also took a certain period of time.
The storm runoff pollution mainly affected the seawater quality and eutrophication in the estuary area and the inner part of the bay, while the outer part of the bay was essentially free from the influence of pollution by storm runoff.
This research was supported by the Project of Technology & Innovation Commission of Shenzhen Municipality (ZDSYS20140509094114169). Hongliang Xu and Ying Zhang are co-first authors of the article.
Supplementary data
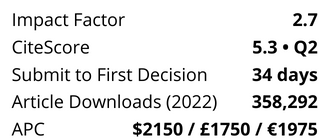
Affiliations

- ISSN 1998-9563 EISSN 2224-7955
- Open Access
- Collections
- Subscriptions
- Subscribe to Open
- Editorial Services
- Rights and Permissions
- Sign Up for Our Mailing List
- IWA Publishing
- Republic – Export Building, Units 1.04 & 1.05
- 1 Clove Crescent
- London, E14 2BA, UK
- Telephone: +44 208 054 8208
- Fax: +44 207 654 5555
- IWAPublishing.com
- IWA-network.org
- IWA-connect.org
- Cookie Policy
- Terms & Conditions
- Get Adobe Acrobat Reader
- ©Copyright 2024 IWA Publishing
This Feature Is Available To Subscribers Only
Sign In or Create an Account
Europe PMC requires Javascript to function effectively.
Either your web browser doesn't support Javascript or it is currently turned off. In the latter case, please turn on Javascript support in your web browser and reload this page.
Search life-sciences literature (44,154,865 articles, preprints and more)
- Available from publisher site using DOI. A subscription may be required. Full text
- Citations & impact
- Similar Articles
Assessment and management of lake eutrophication: A case study in Lake Erhai, China.
Author information, affiliations, orcids linked to this article.
- Shen SL | 0000-0002-5610-7988
- Lin SS | 0000-0002-9120-2040
The Science of the Total Environment , 16 Aug 2020 , 751: 141618 https://doi.org/10.1016/j.scitotenv.2020.141618 PMID: 33167190
Abstract
Full text links .
Read article at publisher's site: https://doi.org/10.1016/j.scitotenv.2020.141618
Citations & impact
Impact metrics, citations of article over time, article citations, eco-environmental changes due to human activities in the erhai lake basin from 1990 to 2020..
Liu X , Chen J , Tang BH , He L , Xu Y , Yang C
Sci Rep , 14(1):8646, 15 Apr 2024
Cited by: 0 articles | PMID: 38622188 | PMCID: PMC11018612
Effective microorganism water treatment method for rapid eutrophic reservoir restoration.
Tomczyk P , Wierzchowski PS , Dobrzyński J , Kulkova I , Wróbel B , Wiatkowski M , Kuriqi A , Skorulski W , Kabat T , Prycik M , Gruss Ł , Drobnik J
Environ Sci Pollut Res Int , 31(2):2377-2393, 08 Dec 2023
Cited by: 0 articles | PMID: 38066279 | PMCID: PMC10791712
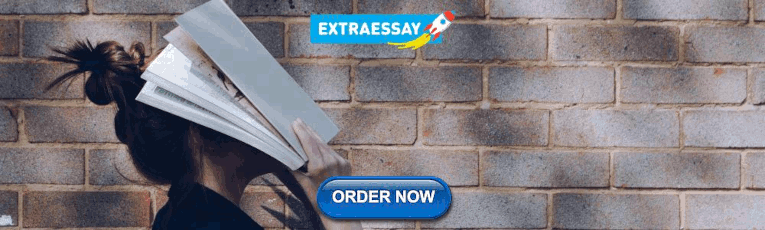
Relationships between Legionella and Aeromonas spp. and associated lake bacterial communities across seasonal changes in an anthropogenic eutrophication gradient.
Grabowska-Grucza K , Kiersztyn B
Sci Rep , 13(1):17076, 10 Oct 2023
Cited by: 0 articles | PMID: 37816753 | PMCID: PMC10564844
Spatio-temporal evolution of eutrophication and water quality in the Turawa dam reservoir, Poland.
Buta B , Wiatkowski M , Gruss Ł , Tomczyk P , Kasperek R
Sci Rep , 13(1):9880, 19 Jun 2023
Cited by: 1 article | PMID: 37336929 | PMCID: PMC10279673
Metagenomic Analysis Reveals the Response of Microbial Communities and Their Functions in Lake Sediment to Environmental Factors.
Song D , Huo T , Zhang Z , Cheng L , Wang L , Ming K , Liu H , Li M , Du X
Int J Environ Res Public Health , 19(24):16870, 15 Dec 2022
Cited by: 1 article | PMID: 36554758 | PMCID: PMC9779402
Similar Articles
To arrive at the top five similar articles we use a word-weighted algorithm to compare words from the Title and Abstract of each citation.
Eutrophication forcings on a peri-urban lake ecosystem: Context for integrated watershed to airshed management.
Putt AE , MacIsaac EA , Herunter HE , Cooper AB , Selbie DT
PLoS One , 14(7):e0219241, 24 Jul 2019
Cited by: 4 articles | PMID: 31339893 | PMCID: PMC6655610
Review of characterization, factors, impacts, and solutions of Lake eutrophication: lesson for lake Tana, Ethiopia.
Ayele HS , Atlabachew M
Environ Sci Pollut Res Int , 28(12):14233-14252, 30 Jan 2021
Cited by: 9 articles | PMID: 33517530
Effects of basin nutrient discharge variations coupled with climate change on water quality in Lake Erhai, China.
Zhang J , Zhi M
Environ Sci Pollut Res Int , 27(35):43700-43710, 01 Aug 2020
Cited by: 0 articles | PMID: 32740833
Quantitative Assessment of Water Quality Improvement by Reducing External Loadings at Lake Erhai, Southwest China.
Gong F , Luo L , Li H , Chen L , Zhang R , Wu G , Zhang J , Shi W , Zhang F , Zhang H , Sun T
Int J Environ Res Public Health , 20(6):5038, 13 Mar 2023
Cited by: 0 articles | PMID: 36981948 | PMCID: PMC10048958
Eutrophication of lake waters in China: cost, causes, and control.
Le C , Zha Y , Li Y , Sun D , Lu H , Yin B
Environ Manage , 45(4):662-668, 23 Feb 2010
Cited by: 80 articles | PMID: 20177679
Funding
Funders who supported this work.
Government of Guangdong Province
Shantou university.
Europe PMC is part of the ELIXIR infrastructure

EnvironBuzz™ Magazine
Communicating Sustainability

The Issue of Eutrophication: A Case Study of Lake Erie
Have you ever encountered a green water body with an abundance of algae floating on the surface? That is a significant symptom of eutrophication. An increasing number of lakes and water bodies look like this globally, including some of our most crucial bodies of water.
What is Eutrophication?
Eutrophication is a process by which excess nutrients such as phosphorus (P) and nitrogen (N) deposit into a body of water and become concentrated in particular areas. This process disturbs natural systems and often causes adverse effects. While eutrophication is a natural process in aging lakes, human activities accelerate this process in younger waters by depositing excess nutrients (Castro & Freitas, 2010). Some human activities that drive this process include population growth; urbanization; industrial expansion; agricultural pollution; water supply development; and changes in land use.
These areas of high nutrients often experience accelerated algae growth, resulting in algae blooms . These blooms disturb the aesthetic nature of the water bodies and impact water oxygen levels. As these algae blooms begin to spread, they quickly take in the oxygen from the water, decreasing oxygen levels. With lower oxygen levels, the fish populations become trapped in hypoxic (deficient levels of oxygen) waters leading to their mortality, also known as fish kills . These hypoxic areas in the waterbody are also called dead zones . These dead zones are areas where the eutrophication process is causing such low oxygen levels that nothing can survive in these areas.
In addition to causing biodiversity loss, eutrophication also impacts human health and the economy. This process reduces water quality, causing it to become undrinkable. Some toxins are irritants, and some are suspected to be carcinogens . The fish in these lakes can also become inedible and sometimes toxic, thus affecting the livelihoods of the local communities.
Eutrophication and Climate Change
Climate change has also had a hand in accelerating eutrophication. Changes in climates and temperatures impact the natural cycle and processes of water bodies, leading to “ significant changes in the physical structure and the biological configuration of the waters .” Eutrophication processes often thrive in warmer, nutrient-rich areas with prolonged ice-free seasons. So as climate change prevails, the warmer season lengthens in places with colder winters, such as Canada. As the warmer periods in a year extend, so does the growing period for these plants and toxins.
A Case Study of Lake Erie
Lake Erie is the world’s eleventh-largest lake in terms of surface area and is situated on the international boundary between Canada and the United States. It “ supplies drinking water to 11 million [people, contains] 50% of the fish found in all of the Great Lakes combined ,” and is home to numerous aquatic species.
It is the southernmost, warmest, and shallowest of the Great Lakes, making it the perfect breeding ground for eutrophication. Because of this, eutrophication is nothing new to Lake Erie, as concerns began in the 1960s and 1970s when increased phosphorus inputs from various human activities resulted in a notable degradation in water quality. In response, in 1972, phosphorus abatement programs were initiated as part of the Great Lakes Water Quality Agreement , which had quick success in Erie and reigned till 1987. But the declaration of the ‘restoration’ of Lake Erie quickly reversed , and algae populations have continued to increase and affect the lake since the mid-1990s and have caused excessive oxygen depletion.
The current eutrophication of Lake Erie threatens all the services provided by this lake. Consequently, gaining a handle on this issue will not only help sustain the services currently offered by the lake but will also enhance the potential for future services.
Solutions to Eutrophication
As part of any remediation project, further research into reliable indicators of eutrophication and direct indicators of the sources of these nutrients is necessary to manage these ecosystems.
Understanding which nutrients is essential for mitigation is also crucial to successful management. Research has concluded a unanimous agreement that reducing phosphorus inputs will directly benefit eutrophication reduction. However, there is a gap in research about nitrogen’s role in eutrophication, as reducing nitrogen alone will not aid mitigation. Research into reducing phosphorus and nitrogen inputs together is still needed before understanding the optimal course-of-action.
There are many innovative approaches to solving eutrophication. An example of a solution to the source of of the issue is vertical farming . Vertical farming is an excellent alternative to intensive agriculture with less input of nutrients. Vertical farming is a closed system that does not deposit nutrients into earth systems.
Another innovative solution to eutrophication is duckweed. Duckweed can be used as a remediation for the aftermath of he process. Duckweed is a plant that has taken nutrients used in agricultural practices and provides oxygen back into the water source. The use of duckweed has the potential to reverse the adverse effects of eutrophication and restore natural ecosystems.
Canada has begun their remediation process for Lake Erie. It recognized the eutrophication of the lake as an ecological risk. Their action plan was published in February 2018 and will start in 2023, with revision every five years after. The plan outlines the importance ; their actions to achieve phosphorus reduction targets ; their efforts to improve policies ; and areas needing more research .
Canada, E. and C. C. (2018, March 13). Government of Canada. Canada.ca. Retrieved December 8, 2022, from https://www.canada.ca/en/environment-climate-change/services/great-lakes-protection/action-plan-reduce-phosphorus-lake-erie.html
Castro, P., & Freitas, H. (2010). Linking anthropogenic activities and eutrophication in estuaries: The need of reliable indicators. Eutrophication: Causes, Consequences and Control, 265–284. https://doi.org/10.1007/978-90-481-9625-8_13
Dokulil, M. T., & Teubner, K. (2010). Eutrophication and climate change: Present situation and future scenarios. Eutrophication: Causes, Consequences and Control, 1–16. https://doi.org/10.1007/978-90-481-9625-8_1
Kane, D. D., Conroy, J. D., Richards, R. P., Baker, D. B., & Culver, D. A. (2014). Re-eutrophication of Lake Erie: Correlations between tributary nutrient loads and phytoplankton biomass. Journal of Great Lakes Research , 40 (3), 496-501. Retrieved from: [PDF] researchgate.net
Landesman, L., Fedler, C., & Duan, R. (2010). Plant nutrient phytoremediation using duckweed. Eutrophication: Causes, Consequences and Control, 341–354. https://doi.org/10.1007/978-90-481-9625-8_17
Sayer, C.D., Davidson, T.A., Rawcliffe, R. et al. Consequences of Fish Kills for Long-Term Trophic Structure in Shallow Lakes: Implications for Theory and Restoration. Ecosystems 19, 1289–1309 (2016). https://doi.org/10.1007/s10021-016-0005-z
Scavia, D., Allan, J. D., Arend, K. K., Bartell, S., Beletsky, D., Bosch, N. S., … & Zhou, Y. (2014). Assessing and addressing the re-eutrophication of Lake Erie: Central basin hypoxia. Journal of Great Lakes Research , 40 (2), 226-246. Retrieved from: Assessing and addressing the re-eutrophication of Lake Erie: Central basin hypoxia – ScienceDirect
Watson, S. B., Miller, C., Arhonditsis, G., Boyer, G. L., Carmichael, W., Charlton, M. N., … & Wilhelm, S. W. (2016). The re-eutrophication of Lake Erie: Harmful algal blooms and hypoxia. Harmful algae , 56 , 44-66. Retrieved from: https://ciglr.seas.umich.edu/wp-content/uploads/2017/09/Watson_etal.pdf.pd f

About Post Author
Sarah lawless.
- Latest Posts

- Grass Lawns: Effects and Alternatives - February 10, 2023
- Leather Alternatives: Sustainable Textile Manufacturing - February 3, 2023
- Sustainable Manufacturing of Personal Products: Toothpaste Case Study - January 27, 2023
Average Rating
Leave a reply cancel reply.
Your email address will not be published. Required fields are marked *
Notify me via e-mail if anyone answers my comment.
Thank you for visiting nature.com. You are using a browser version with limited support for CSS. To obtain the best experience, we recommend you use a more up to date browser (or turn off compatibility mode in Internet Explorer). In the meantime, to ensure continued support, we are displaying the site without styles and JavaScript.
- View all journals
- My Account Login
- Explore content
- About the journal
- Publish with us
- Sign up for alerts
- Open access
- Published: 28 May 2024
Switches in food and beverage product purchases can reduce greenhouse gas emissions in Australia
- Allison Gaines ORCID: orcid.org/0000-0002-2026-2038 1 , 2 ,
- Maria Shahid 2 ,
- Daisy Coyle 2 ,
- Eden Barrett ORCID: orcid.org/0000-0003-0190-6130 2 ,
- Michalis Hadjikakou ORCID: orcid.org/0000-0002-3667-3982 3 ,
- Jason H. Y. Wu ORCID: orcid.org/0000-0003-2073-3562 2 , 4 ,
- Fraser Taylor 2 ,
- Simone Pettigrew ORCID: orcid.org/0000-0003-3921-1174 2 ,
- Bruce Neal 1 , 2 &
- Paraskevi Seferidi 5
Nature Food ( 2024 ) Cite this article
1451 Accesses
1 Citations
687 Altmetric
Metrics details
- Climate-change policy
- Environmental impact
Switching between similar food and beverage products may reduce greenhouse gas emissions (GHGe). Here, using consumer data linked to 23,550 product-specific GHGe values, we estimated annual GHGe attributable to product purchases consumed at home in Australia and calculated reductions from specific switches. Potential changes to mean Health Star Rating, mean energy density and the proportion of ultraprocessed foods purchased were assessed. Approximately 31 million tonnes of GHGe were attributable to products consumed at home in 2019, the three highest contributors of GHGe being ‘meat and meat products’ (49%), ‘dairy’ (17%) and ‘non-alcoholic beverages’ (16%). Switching higher-emission products for ‘very similar’ lower-emission products could reduce total emissions by 26%. Switches to ‘less similar’ lower-emission products could lead to a 71% reduction. Switches had little impact on the average Health Star Rating, energy density of purchases and proportion of ultraprocessed foods purchased. Directing manufacturing and marketing towards lower-environmental-impact products and signposting such options to consumers are key.
Similar content being viewed by others
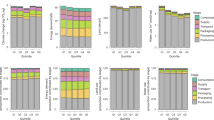
Environmental impacts along the value chain from the consumption of ultra-processed foods
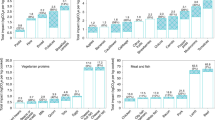
Impacts of home cooking methods and appliances on the GHG emissions of food
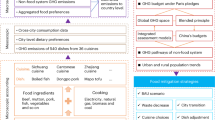
GHG mitigation strategies on China’s diverse dish consumption are key to meet the Paris Agreement targets
The food and beverage sector ranks second only to the energy sector in terms of global contributions to carbon dioxide equivalent greenhouse gas emissions (GHGe) 1 . Food and beverage consumption patterns, particularly in higher-income countries, need to change substantially to become environmentally sustainable according to the Paris Climate Agreement and other international reports 2 , 3 , 4 . In these countries, half to three-quarters of consumption is composed of food and beverage products purchased in supermarkets and other grocery retail outlets 5 , 6 , subsequently making this sector a substantial contributor to overall food and beverage GHGe.
Interventions targeting GHGe attributable to food and beverage products sold in retail stores are limited. The large volume of products available, the insufficient product-specific GHGe data and the complexity of global supply chains all present notable challenges to the development and implementation of effective interventions. Although some existing research describes the environmental impacts of supermarket products 7 , most literature provides GHGe data on broad categories of products and agricultural commodities and no such datasets have been combined with purchase information 8 . Given that supermarket and packaged food and beverage products dominate consumer dietary intake, evidence is needed to inform policy changes (for example, mandatory GHGe labelling of products) that might meaningfully reduce GHGe 9 , 10 .
As we strive towards sustainable food systems, an important objective is to nudge consumers towards purchasing choices that have lower GHGe 11 , 12 . The combination of a product’s carbon footprint and its purchase volume will inform its total contribution to food supply GHGe. It is therefore important to understand purchasing patterns of available products in order to develop targeted policies. A recent study modelling GHGe and other environmental impacts of vegan, vegetarian, fish-eating and low- and high-meat-eating diets highlighted the wide range in impacts within each diet type 13 . Across numerous studies, meat and confectionery product types were found to have marginally higher GHGe per serving, though there was also a wide range in product-specific impacts 7 , 8 , 14 , 15 . Other recent studies realize the potential for feasible product switches to substantially contribute to the reduction in food system emissions required to meet the Paris Climate Agreement goals or achieve net zero for the sector 4 , 16 . Ultimately, environmental food policy should promote products that minimize attributable GHGe and other environmental impacts while also ensuring that health policy targeting better nutrition is not compromised 17 .
In this Analysis, we aimed to utilize product-specific data on the GHGe of Australian food and beverage products (hereon referred to as products) to estimate the potential impact of switching higher-emission products to lower-emission products, within very similar (for example, higher-GHGe white breads switched for lower-GHGe white breads) and less similar categories (for example, higher-GHGe garlic breads to lower-GHGe white breads). We also sought to compare emissions attributable to product purchases across socio-economic groups and quantify the impact of switches on the average nutrient profiling score, level of processing and energy density of purchases.
Grocery purchase information was used from the 2019 NielsenIQ Homescan Consumer Panel, a dataset that captures all product purchases consumed at home by participating Australian households between January and December each year. There were complete data available from 7,535 households that recorded purchases of a total of 14,957,694 products in 2019 (Supplementary Fig 1 ). These data were weighted to represent the total Australian population of 25 million people, with an average of 2.58 (standard deviation (s.d.) 1.42) people per household (Table 1a ).
There were 64,041 different products with unique barcodes included in the NielsenIQ Homescan database, and 22,264 (34.8%) were matched to GHGe data in the 2019 FoodSwitch database. The FoodSwitch database contains product-specific nutritional information 18 , 19 including a product’s nutrient profiling score based on the Health Star Rating (HSR; a front-of-pack summary score developed by the Australian and New Zealand Governments that assigns products between 0.5 stars (least optimal nutrient profile) and 5.0 stars (most optimal nutrient profile) based on a range of nutritional metrics) 20 , level of processing based on the NOVA classification (NOVA; a system that categorizes food and beverage products to one of four groups based on extent and purpose of processing) 21 , 22 and carbon-dioxide-equivalent GHGe estimates 23 for barcoded Australian products. These products accounted for 88.5% of total units purchased (Supplementary Table 1 ). Products included in this study were grouped according to the hierarchical FoodSwitch categorization structure comprising 16 major food categories (for example, ‘bread and bakery products’), 74 minor food categories (for example, ‘bread’) and 703 leaf categories (for example, ‘white bread’). The 41,777 products in the NielsenIQ Homescan database that could not be matched to a FoodSwitch product were distributed approximately evenly across different food and beverage categories, so were consequently omitted from analyses. NielsenIQ categories with the largest number of non-matched products were biscuits (5.00% of non-matched products), breads (4.81%) and snack foods (4.77%).
Estimated GHGe in 2019
Based on the matched purchases, there were 31.3 million (M) tonnes of GHGe attributable to products consumed at home in Australia in 2019. The mean GHGe per person per annum was 1.41 (1.02) tonnes, and the mean GHGe per household per annum was 3.23 (2.24) tonnes (Table 1a ). Households in areas with the greatest disadvantage, defined on the basis of postcode using the Index of Relative Social Advantage and Disadvantage (IRSAD) 24 , 25 , tended to purchase a higher volume of grocery retail products per person per year and therefore contributed significantly higher GHGe per person per annum from this source ( P value <0.05). In addition, these households purchased products with significantly higher average energy density than households in areas with the least disadvantage (Table 1b ; P value <0.05). Average HSR did not vary across household socio-economic position ( P value 0.38).
The three most GHGe-intensive food categories per kilogram of product were ‘meat and meat products’ (6.81 kg GHGe per kilogram), ‘confectionery’ (5.56 kg GHGe per kilogram) and ‘dairy’ (4.14 kg GHGe per kilogram) (Fig. 1 ). After weighting for purchase volumes, the food categories that contributed the most to emissions in 2019 were ‘meat and meat products’ (15.4M tonnes GHGe), ‘dairy’ (5.45M tonnes GHGe) and ‘non-alcoholic beverages’ (5.04M tonnes GHGe; Fig. 1 ). ‘Meat and meat products’ made up 49.0% of total emissions with only 11.2% of total purchases. By contrast, ‘dairy’ and ‘non-alcoholic beverages’, which were ranked third and sixth in terms of kilograms of GHGe per kilogram of product, contributed the second and third largest proportions of total emissions (17.4% and 16.1%, respectively). This was a consequence of their high purchase volumes (21.1% and 20.9% of total purchases, respectively; Supplementary Table 2 ). The predominant contribution of ‘meat and meat products’, ‘dairy’ and ‘non-alcoholic beverages’ to total GHGe in 2019 was apparent not just for the overall population but also for every socio-economic group (Fig. 2 ).
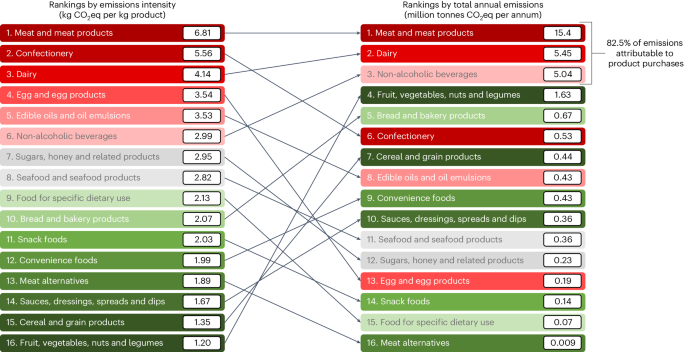
The illustration compares rankings by emissions intensity and total emissions attributable to foods and beverages brought into Australian homes in 2019. Emission intensity data have been published previously 23 .
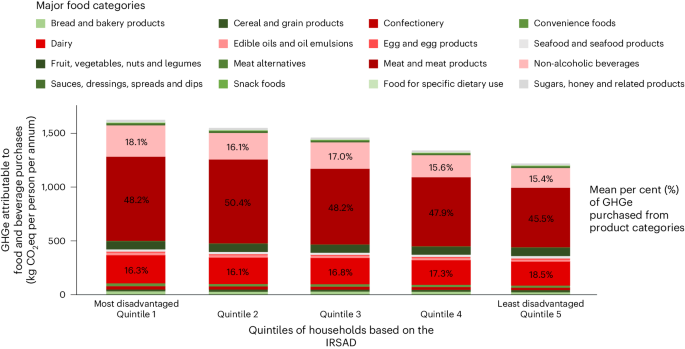
This graph illustrates GHGe representative of products brought into the home per person in Australia in 2019 according to quintiles of socio-economic position. Shading and per cent values indicate proportions of GHGe attributable to different food categories. Intake from restaurants and take-away venues are not considered here. For ease of presentation, any category contributing less than <10% of emissions does not have the percentage listed.
Effects of switching product purchases to reduce GHGe
Switching higher-GHGe products for similar lower-GHGe products showed clear potential for reducing the GHGe attributable to products brought into the home (example product categories presented in Supplementary Table 3 and example product switches presented in Supplementary Table 4 ). For the switches made within the 703 leaf categories, where switched products were very similar to those initially selected, we found potential to decrease total emissions by 26.6% when all products above the 5th percentile of GHGe were replaced; the decrease was only 3.3% when products above the 75th percentile were replaced (Fig. 3a ). For less similar switches within the minor food category, the potential reduction in GHGe was almost three times greater (70.6% and 16.6% when replacing products above the 5th and 75th percentiles, respectively; Fig. 3b ). In general, the greatest absolute and proportional reductions in GHGe all derived from switches made in ‘meat and meat products’, ‘dairy’ and ‘non-alcoholic beverages’ categories (Supplementary Tables 5 and 6 ).
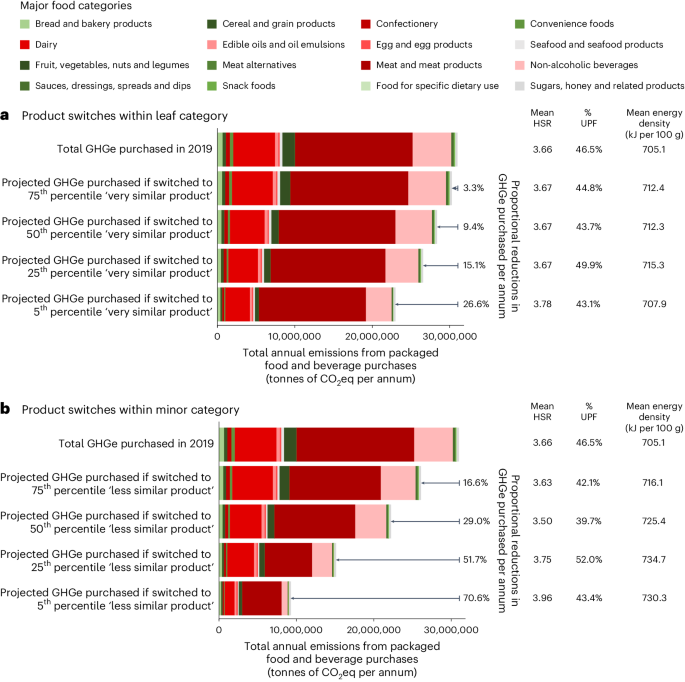
a , b , The graphs illustrate potential reductions in GHGe attributable to foods and beverages brought into the home in Australia in 2019 by making switches to alternative ‘very similar’ products ( a ) and to alternative ‘less similar’ products ( b ). The shading indicates proportions of GHGe attributable to different food categories. The HSR is a score from 0.5 stars (least optimal nutritional profile) to 5.0 stars (most optimal nutritional profile). UPFs are based on the NOVA classification, a system that classifies products as group 1 (minimally processed), group 2 (culinary ingredient), group 3 (processed) or group 4 (ultraprocessed).
Switching purchases to products with lower GHGe had only a small impact on the average HSR and proportion of UPFs of purchased products. For example, the mean HSR of products purchased changed from 3.66 to 3.96 and portion of ultraprocessed foods (UPFs) purchased changed from 46.5% to 43.4% when products with GHGe estimates above the fifth percentile were replaced with less similar lower-emission products (Fig. 3b ). Likewise, there was little impact on the average energy density of purchases when switching to products with lower GHGe (Fig. 3a,b ).
The GHGe attributable to grocery retail purchases in Australia could be greatly reduced by making switches from higher- to lower-emission products. While a modest reduction in GHGe is possible when switching purchases to very similar products, a larger reduction in GHGe could be achieved if switches were made to less similar products within product categories. The plausibility of switching purchases to very similar products is likely to be high, but the consumer acceptability of more substantive switches to less similar products may be lower. The ability to compare products within categories and estimate the potential to reduce annual GHGe from product purchases consumed at home illustrated in these analyses provides a unique opportunity to strengthen Australia’s efforts to reduce national GHGe.
A key finding from the study is that ‘meat and meat products’ contributed to almost 50% of all GHGe attributable to product purchases consumed at home in Australia in 2019, despite accounting for only 11.2% of purchases. The high contribution of ‘meat and meat products’ to the attributable GHGe can be explained by the very high GHGe per kilogram 8 , 14 , 15 . ‘Dairy’ and ‘confectionery’, ranked second and sixth, respectively, were leading contributors of attributable GHGe, driven by their high intensity of emissions per kilogram of product. While ‘dairy’ products are often good sources of nutrients, targeted switching within the category could lead to lower emission purchases. As ‘confectionery’ products are all discretionary, encouraging a lower volume of intake would be most beneficial for this category 26 . ‘Non-alcoholic beverages’, although ranked sixth for emissions intensity, were ranked third by total annual emissions weighted by purchases as a result of their high purchase volumes. The top three product categories are most important to address, as they make up 82.5% of total annual food and beverage emissions weighted by purchases. ‘Fruit, vegetables, nuts and legumes’ have very low GHGe per kilogram of product, so while they are the fourth highest contributor to total GHGe because they make up over one-quarter of total purchases, this amounts to only 5.20% of total attributable emissions.
This study found that households with greater area-level disadvantage tended to bring higher volumes of food and beverage purchases into the home, which is consistent with prior research 27 and corresponds with their higher contribution of attributable GHGe. This is probably because these households tend to purchase a higher proportion of their consumed foods from supermarkets and grocery outlets 28 . Overall, our research found that patterns of consumption, based on the proportion of products purchased per food category, were homogeneous across different household socio-economic positions.
Our study showed that switches made to reduce the GHGe of product purchases did not have a major impact on the average HSR, energy density or proportion of UPFs of product purchases. Previous analyses have reported significant associations between nutrient and energy content, as well as level of processing, and environmental sustainability 29 , 30 , 31 , 32 . However, these have been typically comparing between broad product categories such as meat versus dairy versus bread, where differences in both nutrient profiles and sustainability are likely to be large and easily documented 29 , 30 , 31 , 32 . Our study explored product switches within very similar categories of foods, which were inherently more likely to result in homogeneity of nutrient profiles than homogeneity of environmental sustainability.
International organizations, including the United Nations 33 , recommend that food policies should consider both impacts on human health and environmental sustainability 34 , 35 , highlighting the need to ensure that any recommendations targeting reduction of environmental impacts of diets do not contradict existing nutrition policy recommendations. To remain consistent with such dual policy recommendations, we used HSR, NOVA classification and energy density to explore potential unintended side effects of food switches for sustainability on diet. HSR was used as it is the nutrient profiling model currently used by the governments of Australia and New Zealand 20 to regulate nutrition policy targeting packaged food and beverage products, such as front-of-pack nutrition labelling. It is a standardized and replicable model 36 , similar to nutrient profiling models used in other countries 37 . However, HSR does not holistically capture all aspects of product healthiness 20 , 38 or consider broader food consumption patterns and lacks robust validation 39 , 40 , 41 . In general, nutrient profiling models are limited due to their reliance on food components relative to other similar foods (for example, amounts of specific nutrients) rather than a holistic assessment of diet 42 . In some cases, nutrient profiling models have been found to contribute to a false impression of healthiness due to the presentation of positive nutrient qualities (for example, low in fat or high in protein), the non-presentation of negative nutrient qualities (for example, high in added sugars) and little consideration of other health impact factors including level of processing, a phenomenon termed ‘health halos’ 43 , 44 . As such, the effects on HSR reported in this paper do not fully reflect the impact of making switches on the healthiness of the diet. Thus, we also explored associations between product switches for sustainability and the proportion of UPFs purchased based on product NOVA classification. UPF consumption has been repeatedly associated with adverse health outcomes, such as increased risk of obesity, cardio-metabolic outcomes and mortality 45 , 46 , and the use of the NOVA classification has been shown to improve validity of nutrition classification schemes 47 . By including diverse indicators of diet in our analysis, both currently implemented policy tools, such as HSR, and more holistic classifications, such as NOVA, we provide a rounded overview of the potential impacts of modelled switches on diet, reinforcing the significance of dual action policies and the move away from siloed approaches to food policy 34 .
The joint impact of emissions intensity and purchase volumes on total attributable GHGe highlights the need for urgent policy interventions that target both the most environmentally intensive and the most widely purchased food and beverage products. We found that there is potential for product switches to notably contribute to the reduction in food system emissions required to meet the Paris Climate Agreement goals or achieve net zero for the sector 4 . Further, in all assumed emission-reduction scenarios, most of the potential reduction in GHGe was attributable to three food and beverage categories (that is, ‘meat and meat products’, ‘dairy’ and ‘non-alcoholic beverages’), which identifies them as primary intervention targets. Since total GHGe due to ‘fruit, vegetables, nuts and legumes’ were low compared with the leading three causes of GHGe and their average nutritional quality was high, environmental gains from targeting this and other categories making lesser contributions would be limited. We found only minor variations with respect to purchases of the leading GHGe-contributing food categories for households of different socio-economic position. As such, while the implementation of interventions seeking to reduce GHGe may need to be tailored to suit different socio-economic groups, the target categories for policy intervention (that is, ‘meat and meat products’, ‘dairy’ and ‘non-alcoholic beverages’) should be the same across communities.
Policy intervention options to promote better-for-environment products are not yet being implemented at the industry or consumer level in Australia. In the first instance, Australian policymakers could include the environmental impacts of products into the Australian Dietary Guidelines, following the example of New Zealand 48 . There is also the option to require on-pack labelling of GHGe, similar to the current implementation of the country-of-origin label 49 . While some private carbon-labelling schemes have been implemented on a few products 50 , 51 , the work here would enable broader labelling implementation in the Australian context that could greatly enhance consumer decision-making 52 , 53 . Though potentially the least feasible, taxes could be applied to higher-emission and lower-nutritional-quality products using category-specific limits, as this would promote products that are better for people and the planet, and allow accessible dietary variety across households from different socio-economic positions 54 .
These analyses focused on the effects that food and beverage choices could have on reducing GHGe attributable to household product purchases and understanding the potential to optimize product choices based on environmental sustainability. A different method called substitution analysis, which constrains switches by nutrients, volume and energy, could also be done 55 , 56 , though the minimal differences in the average HSR, energy density and proportion of UPFs purchased of switched products from current purchase patterns suggest that the conclusions from such analyses might be similar. It is also important to note that we did not estimate the potential impacts of product switches on (1) costs, either overall or relative to the purchasing capacity of different socio-economic positions, as the available price data were limited 57 , or (2) other environmental factors (for example, land degradation, scarcity-weighted water use and eutrophication), as these are not yet included in FoodSwitch 23 . While also out of scope for this analysis, future assessment of GHGe reductions by incorporating the frequency of specific product purchases could help to improve both the consumer acceptability and likelihood of product switches. Switching between relevant product categories (for example, ‘meat and meat products’ to ‘meat alternatives’) would be an important next step, as well. These should be considered in future work to enable stakeholders and policymakers to incorporate these impacts into product and policy enhancements. Finally, it will be important to assess the social acceptability of product switches using surveys and behavioural analyses.
This work used product-specific GHGe estimates and household-level purchasing information for an entire food and beverage supply, which provided a unique opportunity to compare emissions both between and within product categories. The NielsenIQ Homescan data are widely considered to provide a valid estimation of products consumed at home, and analyses based on these data have received substantial attention from academia and policymakers 58 , 59 , 60 . They are nationally representative of Australian households by region and socio-economic position and cover almost 90% of total product units purchased yearly. The underlying purchasing data employed here have been used previously to understand the potential health impacts of optimizing nutrient intakes through interventions, such as salt reduction or the implementation of dietary guidelines 61 , 62 . Both the NielsenIQ Homescan and FoodSwitch datasets have been used in multiple prior analyses of product purchasing patterns in Australia and other jurisdictions 58 , 63 , 64 , 65 .
There are limitations to the FoodSwitch database, including incomplete coverage of all products sold in Australia and the requirement for imputation of data that are not reported on pack 18 . The product-specific GHGe estimates use a novel methodology that provides summary results that are well aligned with prior reports 7 , 8 , but there is probably imprecision in the estimates for some individual products. The GHGe estimates include approximations for most ingredients and crudely account for GHGe associated with processing and transport. So far, the estimates do not include geospatial emissions data (that is, global GHGe averages for each ingredient are used), as the source information pertaining to each ingredient is not often available for packaged products. The GHGe associated with refrigeration, packaging and required preparations (for example, cooking or microwaving) are also not currently considered in product estimates.
There are some well-established limitations to the use of NielsenIQ Homescan data for these types of analysis. The absence of information relating to products consumed outside the home (for example, from restaurants and take-away outlets) may lead to an under-estimation of emissions attributable to products purchased across Australia, especially among the least-deprived households. The misreporting of product purchases (for example, omission of products purchased but never brought into the home) may also lead to an overall under-estimation of product emissions across households. Finally, household-specific extrapolation from product purchases to consumption may be miscredited, as purchased products could be shared with, donated or otherwise consumed by persons outside of the household.
In conclusion, large reductions in GHGe attributable to foods and beverages could be achieved through relatively plausible switches in product purchases. There is a clear and immediate opportunity for the Australian government, which is being urged to consider environmental sustainability of diets 66 , to implement policies that nudge consumers and industry towards lower-emission product choices. ‘Meat and meat products’, ‘dairy’ and ‘non-alcoholic beverages’ were identified as the leading causes of GHGe attributable to product purchases consumed at home in Australia and are the three categories where there is clear potential to substantially reduce GHGe 12 , 62 . Intervention options are multiple, and potential priority actions include a requirement for on-pack labelling of GHGe and the exploration of fiscal incentives that will drive industry production practices and consumer purchasing patterns towards more environmentally friendly choices.
In this cross-sectional study, we calculated the annual GHGe attributable to major categories of products purchased from grocery retail outlets and brought into Australian homes in 2019. The study describes variations in food purchases across households of different socio-economic position and ranks the relative contribution of food categories to household GHGe attributable to product purchases. The project was approved by the University of New South Wales Human Research Ethics Committee (approval number HC200244).
Study population
The NielsenIQ Homescan panel includes about 10,000 households that are surveyed to be representative of the demographic composition and geographic distribution of the Australian population. Data are collected by householders using handheld electronic scanners to record the barcodes of products purchased from all retail outlet types and brought into the home (that is, does not include items that are eaten elsewhere) 27 , 59 , 60 . In addition, 284 types of individual products without a barcode (such as unpackaged fruits and vegetables) are recorded by householders scanning the applicable barcode from a list provided by NielsenIQ in booklet form.
The dataset contains information on the characteristics of the households, including the number of household members, number of children and area-level socio-economic position. For assessment across socio-economic positions, the IRSAD system ranks geographic areas using a range of indicators including education, income, occupation and housing. Using the information collected in the latest Australian Bureau of Statistics Household Income and Wealth survey 25 , household areas were divided into quintiles, with quintile 1 representing the most disadvantaged and quintile 5 representing the least disadvantaged.
Food and beverage data
The 2019 Australian FoodSwitch Annual database used in this analysis contains packaged food and beverage data that have been described in detail previously 18 . Data for the 23,550 included products were obtained by trained data collectors who visited five major supermarket chains in metropolitan Sydney (Coles, Woolworths, IGA, Aldi and Harris Farm) between August and November 2019. Standard and rigorous checks are applied across all products to ensure data quality 67 . Products are organized into main categories (for example, ‘meat and meat products’), then finer minor categories (for example, ‘processed meat’) and subcategories (for example, ‘meat pies’ and ‘coated/breaded frozen/chilled meat’). These categories were derived from a system originally developed by the Global Food Monitoring Group 67 . Products from 16 major food and beverage categories were included (Supplementary Table 3 ). Products from the ‘alcoholic beverages’ and ‘vitamins and supplements’ categories were not included in the current analysis because they are not included in the scope of the HSR.
The NielsenIQ Homescan purchase data were linked to the 2019 FoodSwitch database in order to assess product nutrition information, HSR, NOVA and GHGe. The product-specific GHGe values were estimated primarily on the basis of ingredient life cycle assessment data, using the cradle-to-farm gate system boundary, and adjusted for ingredient processing and ingredient refuse. The ingredient GHGe values were then weighted by ingredient proportions and summed to estimate the product-specific GHGe value, with additional adjustments applied to account for product processing and transport 23 .
Products were matched in a three-step process. First, we aimed to match as many products as possible by their unique barcodes. Products without barcode information were matched according to product name using previously described methods 27 , 68 . We then matched products using their exact product name, and finally using their product name after removing any nutritionally irrelevant descriptors (for example, container type or product shape). The 284 unpackaged, unbarcoded NielsenIQ products were assigned the average GHGe for the closest corresponding leaf category in the FoodSwitch database.
The primary outcome for these analyses was the estimated tonnes of GHGe attributable to product purchases consumed at home in Australia in 2019. This was estimated by totalling the GHGe for each matched product based on the quantity (weight) purchased over the year to obtain an annual GHGe value for product purchases made by each household for each product. The secondary outcomes were the average nutrient profiling score as defined by the mean HSR, the mean energy density and proportion of UPFs purchased as defined by the NOVA group 4 classification, recorded in kJ per 100 g, of products purchased.
Statistical analysis
All results were weighted to represent the overall Australian population using survey sample weights based on the latest Australian Bureau of Statistics census data provided by NielsenIQ Homescan 24 . To control for under-reporting of purchases, households were included if they recorded at least one barcode per week for at least 50% of the 52-week period and spent an average of at least $5 per week on product purchases. We described characteristics and purchasing patterns of included households both for the overall population and across quintiles based on the IRSAD. Differences across quintiles were assessed using one-way analysis of variance (ANOVA). We also described the GHGe contribution of purchases across the 16 major food categories by socio-economic position.
We ranked each of the major food categories, first according to emissions per kilogram of product (that is, kilogram GHGe per kilogram of product) and then according to the total emissions attributable to annual purchases of that product (that is, tonnes GHGe purchased per year). This allowed us to explore the changes in ranking of product categories when weighted by purchases.
We tested the potential impact on GHGe, mean HSR, mean energy density (in kJ per 100 g) and proportion of UPFs purchased (%) of switching product purchases in two ways. First, we examined the impact of making small changes to initial purchasing patterns by switching products within leaf categories ( n = 703), which include very similar products that could be easily switched and are most similar in the functional sense, such as use and contribution to the diet (for example, higher-GHGe white bread products switched for lower-GHGe white bread products). See Supplementary Table 3 for more examples. To estimate the effect of product switching, we determined the 75th percentile GHGe value within each leaf category. We then replaced the GHGe, HSR, energy density values and NOVA group classification of all purchased products with a GHGe value above the 75th percentile with the GHGe, HSR, energy density values and NOVA group classification of the product at the 75th percentile. In turn, we repeated this process for the products lying above the 50th, 25th and 5th percentiles of GHGe to test the impact of progressively switching purchases towards those products with the lowest GHGe 4 , 29 . Next, we repeated this process to examine the potential impact of switching products within the broader minor food categories ( n = 74) of less similar products that maintain less but still some likeliness in functionality (for example, from ‘garlic bread’ products to ‘white bread’ products; see Supplementary Table 3 for more examples). Potential impacts are presented overall and by the 16 major food categories.
All data preparation and statistical analyses were conducted using Stata BE 17 (Stata Corp) and Excel. A two-sided P value <0.05 was considered statistically significant.
Reporting summary
Further information on research design is available in the Nature Portfolio Reporting Summary linked to this article.
Data availability
The Ecoinvent 3.7.1 and Agri-footprint 5.0 databases contained in SimaPro were used to assign ingredient GHGe values. The product-specific source data obtained from the FoodSwitch database are proprietary and sample data can only be made available upon request.
Code availability
The code will be made available upon reasonable request.
Rosenzweig, C. et al. Climate change responses benefit from a global food system approach. Nat. Food 1 , 94–97 (2020).
Article PubMed Google Scholar
Willett, W. et al. Food in the Anthropocene: the EAT–Lancet Commission on healthy diets from sustainable food systems. Lancet 393 , 447–492 (2019).
Springmann, M. et al. Options for keeping the food system within environmental limits. Nature 562 , 519–525 (2018).
Article ADS CAS PubMed Google Scholar
Costa, C. et al. Roadmap for achieving net-zero emissions in global food systems by 2050. Sci. Rep. 12 , 15064 (2022).
Article ADS PubMed PubMed Central Google Scholar
Popkin, B. M. The nutrition transition: an overview of world patterns of change. Nutr. Rev. 62 , S140–S143 (2004).
Popkin, B. M. The nutrition transition and obesity in the developing world. J. Nutr. 131 , 871s–873ss (2001).
Article CAS PubMed Google Scholar
Clark, M. et al. Estimating the environmental impacts of 57,000 food products. Proc. Natl Acad. Sci. USA 119 , e2120584119 (2022).
Article CAS PubMed PubMed Central Google Scholar
Poore, J. & Nemecek, T. Reducing food’s environmental impacts through producers and consumers. Science 360 , 987–992 (2018).
Clément, C. W. Copping out on food systems: how COP26 failed to address food and climate and how COP27 can solve it. J. Agric. Environ. Ethics 35 , 20 (2022).
Article PubMed PubMed Central Google Scholar
Kennedy, E. et al. Transforming food systems: the missing pieces needed to make them work. Curr. Dev. Nutr. 5 , nzaa177 (2021).
Heller, M. C., Keoleian, G. A. & Willett, W. C. Toward a life cycle-based, diet-level framework for food environmental impact and nutritional quality assessment: a critical review. Environ. Sci. Technol. 47 , 12632–12647 (2013).
Swinburn, B. A. et al. The global syndemic of obesity, undernutrition, and climate change: the Lancet Commission report. Lancet 393 , 791–846 (2019).
Scarborough, P. et al. Vegans, vegetarians, fish-eaters and meat-eaters in the UK show discrepant environmental impacts. Nat. Food 4 , 565–574 (2023).
Heller, M. C., Willits-Smith, A., Meyer, R., Keoleian, G. A. & Rose, D. Greenhouse gas emissions and energy use associated with production of individual self-selected US diets. Environ. Res. Lett. 13 , 044004 (2018).
Clune, S., Crossin, E. & Verghese, K. Systematic review of greenhouse gas emissions for different fresh food categories. J. Clean. Prod. 140 , 766–783 (2017).
Article CAS Google Scholar
Grummon, A. H., Lee, C. J. Y., Robinson, T. N., Rimm, E. B. & Rose, D. Simple dietary substitutions can reduce carbon footprints and improve dietary quality across diverse segments of the US population. Nat. Food https://doi.org/10.1038/s43016-023-00864-0 (2023).
Godfray, H. C. J. et al. Meat consumption, health, and the environment. Science 361 , eaam5324 (2018).
Dunford, E. et al. FoodSwitch: a mobile phone app to enable consumers to make healthier food choices and crowdsourcing of national food composition data. JMIR mHealth uHealth 2 , e37 (2014).
Dunford, E. K. & Neal, B. FoodSwitch and use of crowdsourcing to inform nutrient databases. J. Food Compos. Anal. 64 , 13–17 (2017).
Article Google Scholar
Health Star Rating System 2020. Australian Government (2020); http://www.healthstarrating.gov.au/internet/healthstarrating/publishing.nsf/content/home
Monteiro, C., Cannon, G., Levy, R., Moubarac, J.-C. & Jaime, P. NOVA. The star shines bright. Position paper 2. World Nutr. 7 , 28–38 (2016).
Google Scholar
Monteiro, C. A., Cannon, G., Lawrence, M., Louzada, M. L. D. C. & Machado, P. P. Ultra-processed Foods, Diet Quality, and Health Using the NOVA Classification System (FAO, 2019).
Gaines, A. M. et al. A novel approach to estimate product-specific greenhouse gas emissions for 23,550 Australian packaged foods and beverages. J. Clean. Prod. 425 , 138816 (2023).
2016 Census Australia. Australian Bureau of Statistics (ABS, 2023); https://www.abs.gov.au/websitedbs/censushome.nsf/home/2016
6523.0 - Household Income and Wealth Australia. Australian Bureau of Statistics (ABS, 2023); https://www.abs.gov.au/AUSSTATS/[email protected]/Lookup/6523.0Main+Features12015-16?OpenDocument
Hadjikakou, M. Trimming the excess: environmental impacts of discretionary food consumption in Australia. Ecol. Econ. 131 , 119–128 (2016).
Coyle, D. H. et al. Contribution of major food companies and their products to household dietary sodium purchases in Australia. Int. J. Behav. Nutr. Phys. Act. 17 , 81 (2020).
Pechey, R. & Monsivais, P. Supermarket choice, shopping behavior, socioeconomic status, and food purchases. Am. J. Prevent. Med. 49 , 868–877 (2015).
Springmann, M. et al. Health and nutritional aspects of sustainable diet strategies and their association with environmental impacts: a global modelling analysis with country-level detail. Lancet Planet. Health 2 , e451–e461 (2018).
Rippin, H. L. et al. Variations in greenhouse gas emissions of individual diets: associations between the greenhouse gas emissions and nutrient intake in the United Kingdom. PLoS ONE 16 , e0259418 (2021).
Vellinga, R. E. et al. Different levels of ultraprocessed food and beverage consumption and associations with environmental sustainability and all-cause mortality in EPIC-NL. Am. J. Clin. Nutr. 118 , 103–113 (2023).
Kesse-Guyot, E. et al. Environmental impacts along the value chain from the consumption of ultra-processed foods. Nat. Sustain. 6 , 192–202 (2023).
About the Sustainable Development Goals. United Nations (2023); https://www.un.org/sustainabledevelopment/sustainable-development-goals/
Friel, S., Barosh, L. J. & Lawrence, M. Towards healthy and sustainable food consumption: an Australian case study. Public Health Nutr. 17 , 1156–1166 (2014).
Springmann, M. et al. Mitigation potential and global health impacts from emissions pricing of food commodities. Nat. Clim. Change 7 , 69–74 (2017).
Article ADS Google Scholar
Pan, X.-F. et al. Seventeen-year associations between diet quality defined by the health star rating and mortality in Australians: The Australian Diabetes, Obesity and Lifestyle Study (AusDiab). Curr. Dev. Nutr. 4 , nzaa157 (2020).
Mozaffarian, D. et al. Food Compass is a nutrient profiling system using expanded characteristics for assessing healthfulness of foods. Nat. Food 2 , 809–818 (2021).
Azaïs-Braesco, V., Goffi, C. & Labouze, E. Nutrient profiling: comparison and critical analysis of existing systems. Public Health Nutr. 9 , 613–622 (2006).
Scrinis, G. Nutritionism: The Science and Politics of Dietary Advice 1st edn, 362 (Routledge, 2013).
Dickie, S., Woods, J. L., Baker, P., Elizabeth, L. & Lawrence, M. A. Evaluating nutrient-based indices against food- and diet-based indices to assess the health potential of foods: how does the Australian Health Star Rating System perform after five years? Nutrients 12 , 1463 (2020).
Cooper, S. L., Pelly, F. E. & Lowe, J. B. Assessment of the construct validity of the Australian Health Star Rating: a nutrient profiling diagnostic accuracy study. Eur. J. Clin. Nutr. 71 , 1353–1359 (2017).
Cooper, S. L., Pelly, F. E. & Lowe, J. B. Construct and criterion-related validation of nutrient profiling models: a systematic review of the literature. Appetite 100 , 26–40 (2016).
Sütterlin, B. & Siegrist, M. Simply adding the word ‘fruit’ makes sugar healthier: the misleading effect of symbolic information on the perceived healthiness of food. Appetite 95 , 252–261 (2015).
Whalen, R., Harrold, J., Child, S., Halford, J. & Boyland, E. The health halo trend in UK television food advertising viewed by children: the rise of implicit and explicit health messaging in the promotion of unhealthy foods. Int. J. Environ. Res. Public Health 15 , 560 (2018).
Schnabel, L. et al. Association between ultraprocessed food consumption and risk of mortality among middle-aged adults in France. JAMA Intern. Med. 179 , 490–498 (2019).
Elizabeth, L., Machado, P., Zinöcker, M., Baker, P. & Lawrence, M. Ultra-processed foods and health outcomes: a narrative review. Nutrients 12 , 1955 (2020).
Dickie, S., Woods, J., Machado, P. & Lawrence, M. A novel food processing-based nutrition classification scheme for guiding policy actions applied to the Australian food supply. Front. Nutr. 10 , 1–15 (2023).
Eating and Activity Guidelines for New Zealand Adults 2020. Manatū Hauora Ministry of Health (2023); https://www.health.govt.nz/system/files/documents/publications/eating-activity-guidelines-new-zealand-adults-updated-2020-oct22.pdf
Country of origin labelling. Australian Government Department of Industry, Science and Resources (2023); https://www.industry.gov.au/country-origin-labelling
Vandenbergh, M. P., Dietz, T. & Stern, P. C. Time to try carbon labelling. Nat. Clim. Change 1 , 4–6 (2011).
Grunert, K. G., Hieke, S. & Wills, J. Sustainability labels on food products: consumer motivation, understanding and use. Food Policy 44 , 177–189 (2014).
Brown, K. A., Harris, F., Potter, C. & Knai, C. The future of environmental sustainability labelling on food products. The Lancet Planet. Health 4 , e137–e138 (2020).
Miranda-Ackerman, M. A. & Azzaro-Pantel, C. Extending the scope of eco-labelling in the food industry to drive change beyond sustainable agriculture practices. J. Environ. Manage. 204 , 814–824 (2017).
Faccioli, M. et al. Combined carbon and health taxes outperform single-purpose information or fiscal measures in designing sustainable food policies. Nat. Food 3 , 331–340 (2022).
Song, M. & Giovannucci, E. Substitution analysis in nutritional epidemiology: proceed with caution. Eur. J. Epidemiol. 33 , 137–140 (2018).
Ibsen, D. B. et al. Food substitution models for nutritional epidemiology. Am. J. Clin. Nutr. 113 , 294–303 (2020).
Einav, L., Leibtag, E. & Nevo, A. On the Accuracy of Nielsen Homescan Data. Contract No.: 69 (United States Department of Agriculture, 2008).
Lacko, A. M., Guilkey, D., Popkin, B. & Ng, S. W. Associations among select state policies and the nutritional quality of household packaged food purchases in the United States from 2008 through 2017. J. Acad. Nutr. Diet 122 , 731–44.e32 (2022).
Coyle, D. H. et al. Estimating the potential impact of the Australian government’s reformulation targets on household sugar purchases. Int. J. Behav. Nutr. Phys. Act. 18 , 138 (2021).
Coyle, D. et al. Estimating the potential impact of Australia’s reformulation programme on households’ sodium purchases. BMJ Nutr. Prevent. Health 4 , 49–58 (2021).
Trieu, K. et al. Salt reduction initiatives around the world—a systematic review of progress towards the global target. PLoS ONE 10 , e0130247-e (2015).
Springmann, M. et al. The healthiness and sustainability of national and global food based dietary guidelines: modelling study. Br. Med. J. 370 , m2322 (2020).
Poti, J. M., Dunford, E. K. & Popkin, B. M. Sodium reduction in US households’ packaged food and beverage purchases, 2000 to 2014. JAMA Intern. Med. 177 , 986–994 (2017).
Dunford, E. K. & Poti, J. M. Simulating the impact of sodium reduction from packaged foods on population sodium intake in US adults and children. Public Health Nutr. 23 , 488–495 (2020).
Davies, B. & Worrall, S. Basket analysis: profiling British customers. Br. Food J. 100 , 102–109 (1998).
Hendrie, G. A. et al. Towards healthier and more sustainable diets in the Australian context: comparison of current diets with the Australian Dietary Guidelines and the EAT–Lancet Planetary Health Diet. BMC Public Health 22 , 1939 (2022).
Dunford, E. et al. International collaborative project to compare and monitor the nutritional composition of processed foods. Eur. J. Prev. Cardiol. 19 , 1326–1332 (2012).
Slining, M. M., Ng, S. W. & Popkin, B. M. Food companies’ calorie-reduction pledges to improve U.S. diet. Am. J. Prev. Med. 44 , 174–184 (2013).
Download references
Acknowledgements
A.G. acknowledges support for this work by Imperial College London’s President’s PhD Scholarship. M.S. acknowledges funding from Marlow Foods Ltd for a PhD research scholarship. No funding sources were involved in the design, data collection, analysis or interpretation of data in this manuscript.
Author information
Authors and affiliations.
Department of Epidemiology and Biostatistics, School of Public Health, Imperial College London, London, UK
Allison Gaines & Bruce Neal
The George Institute for Global Health, Sydney, New South Wales, Australia
Allison Gaines, Maria Shahid, Daisy Coyle, Eden Barrett, Jason H. Y. Wu, Fraser Taylor, Simone Pettigrew & Bruce Neal
School of Life and Environmental Sciences, Centre for Integrative Ecology, Deakin University, Melbourne, Victoria, Australia
Michalis Hadjikakou
School of Public Health, UNSW Sydney, Sydney, New South Wales, Australia
Jason H. Y. Wu
Public Health Policy Evaluation Unit, School of Public Health, Imperial College London, London, UK
Paraskevi Seferidi
You can also search for this author in PubMed Google Scholar
Contributions
A.G., B.N. and P.S. conceptualized and designed the study. A.G. and M.S. were involved in the data collection. A.G. conducted the analyses for the study, with secondary review from M.S., D.C., F.T., P.S. and B.N. M.S., D.C., E.B., M.H. and J.H.Y.W. verified the accuracy and application of the data. A.G., P.S. and B.N. drafted the paper. A.G., M.S., D.C., E.B., F.T. and B.N. had access to and interpreted the data. All authors read the final paper, reviewed it for important intellectual content and approved its submission.
Corresponding author
Correspondence to Allison Gaines .
Ethics declarations
Competing interests.
The authors declare no competing interests.
Peer review
Peer review information.
Nature Food thanks Patricia Eustachio Colombo, Joe Kennedy and the other, anonymous, reviewer(s) for their contribution to the peer review of this work.
Additional information
Publisher’s note Springer Nature remains neutral with regard to jurisdictional claims in published maps and institutional affiliations.
Supplementary information
Supplementary information.
Supplementary Tables 1–6 and Fig. 1.
Reporting Summary
Rights and permissions.
Open Access This article is licensed under a Creative Commons Attribution 4.0 International License, which permits use, sharing, adaptation, distribution and reproduction in any medium or format, as long as you give appropriate credit to the original author(s) and the source, provide a link to the Creative Commons licence, and indicate if changes were made. The images or other third party material in this article are included in the article’s Creative Commons licence, unless indicated otherwise in a credit line to the material. If material is not included in the article’s Creative Commons licence and your intended use is not permitted by statutory regulation or exceeds the permitted use, you will need to obtain permission directly from the copyright holder. To view a copy of this licence, visit http://creativecommons.org/licenses/by/4.0/ .
Reprints and permissions
About this article
Cite this article.
Gaines, A., Shahid, M., Coyle, D. et al. Switches in food and beverage product purchases can reduce greenhouse gas emissions in Australia. Nat Food (2024). https://doi.org/10.1038/s43016-024-00971-6
Download citation
Received : 13 February 2023
Accepted : 05 April 2024
Published : 28 May 2024
DOI : https://doi.org/10.1038/s43016-024-00971-6
Share this article
Anyone you share the following link with will be able to read this content:
Sorry, a shareable link is not currently available for this article.
Provided by the Springer Nature SharedIt content-sharing initiative
This article is cited by
Challenges associated with greenhouse gas emissions-related food guidance.
- Mark Lawrence
Nature Food (2024)
Quick links
- Explore articles by subject
- Guide to authors
- Editorial policies
Sign up for the Nature Briefing: Anthropocene newsletter — what matters in anthropocene research, free to your inbox weekly.

Eutrophication
Excessive nutrients in a body of water, often leading to algal blooms or other adverse effects.
See also algal bloom.
- Free Samples
- Premium Essays
- Editing Services Editing Proofreading Rewriting
- Extra Tools Essay Topic Generator Thesis Generator Citation Generator GPA Calculator Study Guides Donate Paper
- Essay Writing Help
- About Us About Us Testimonials FAQ
- Studentshare
- Governance Challenges on Eutrophication in Australia
Governance Challenges on Eutrophication in Australia - Case Study Example
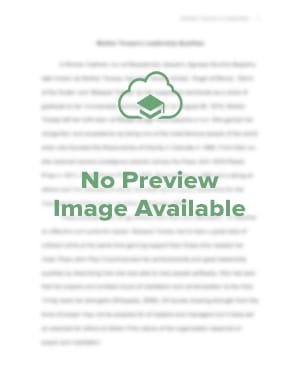
- Subject: Management
- Type: Case Study
- Level: Undergraduate
- Pages: 9 (2250 words)
- Downloads: 0
Extract of sample "Governance Challenges on Eutrophication in Australia"
- Cited: 0 times
- Copy Citation Citation is copied Copy Citation Citation is copied Copy Citation Citation is copied
CHECK THESE SAMPLES OF Governance Challenges on Eutrophication in Australia
The process of eutrophication and coastal eutrophication problem, spirit of the new european maritime policy, climate change and governance of water, governance review of metroland australia limited, governance in australian public sector, governance and accountability in australia, governance challenges in rich countries, securing the marine waters.
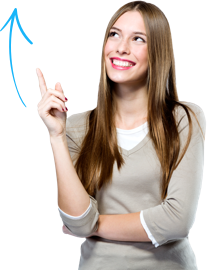
- TERMS & CONDITIONS
- PRIVACY POLICY
- COOKIES POLICY
Many blame social media for poor mental health among teenagers, but the science is murky
If Jordy had a switch to instantly shut down social media, she would flip it.
"I'd switch it off, 100 per cent, even if it was for a week, just so people could have that taste of what it would be like," she said.
Now in her first year out of school, the 18-year-old studies nursing at university and works at a local cafe in Charleville, a small town 745 kilometres west of Brisbane, where she has lived most of her life.
Like Australian teenagers everywhere, she has another life online.
"It's like a second world, really," she said.
"You have reality and then you have social media — two extremely different things."
At the moment, she spends an average of five-and-a-half hours a day on her phone, but it's lower than her peak during high school.
"When I first got a phone I was on it constantly, probably like seven hours, eight hours a day," she said.
She has cut back since then because that second world was not always kind, especially when it came to body image — and despite the fact her parents were always strict about phone usage.
"Growing up, I've always been a big girl … and a sporty person — I'm pretty healthy," she said.
"But when we see images, it tends to be just very thin, skinny people.
"It can just take you down, with the click of your fingers."
Jordy was also being bullied at school, but social media meant it could happen around the clock, no matter where she was.
"A group of boys at my school had tagged me on TikTok telling me to go kill myself," she said.
"It was just so heartbreaking. I was just like, 'I go to school with you every day, we've never had an issue in the past.' That's probably the worst thing that's happened."
Jordy's mental health was tanking, and she began to withdraw from activities she used to love, like footy training or seeing friends.
"I just felt so scared to talk to my mum … I was just like, 'I don't want my mum to think I'm using social media the wrong way'," she said.
No matter how bad things got, logging off still felt impossible.
"It was like that fear of missing out, I guess. I think that's the addiction thing, right?" she said.
"You sort of just have to be on your phone to socialise."
Does more screen time cause worse mental health in teenagers?
Teen mental health has deteriorated at an accelerating rate in the last two decades — more or less exactly since social media and smartphones started to become widespread in 2007.
For obvious reasons, many people, especially concerned parents, have leapt to the conclusion that tech is the culprit.
But the science is surprisingly murky, even though there is a link — research shows more screen time is associated with higher rates of depression in adolescents.
"What we know about the link is there's a link, and that's pretty much what we know," said Aliza Werner-Seidler, a senior researcher at the Black Dog Institute.
"We have really good correlational data, there is a strong linear relationship, particularly in young girls.
"What we don't know is about causation — so is young people's mental health leading them to spend more time on social media and screens, or is it actually the other way around?
"We don't know the direction of the effect."
Dr Werner-Seidler is one of thousands of researchers around the world trying to solve that mystery.
Even if many people are convinced they already know the answer because of their own experience online.
"Personally I would say that it's both," Jordy said.
After a session doomscrolling perfect bodies on TikTok, she "would feel horrible" about herself.
"But then I'd continue to use it and then it made me feel even worse."
After 17 years, why don't we have the answers yet?
Despite 17 years of widespread smartphones and social media, researchers still don't have enough data to definitively say whether they're to blame for deteriorating teen mental health.
Getting those long-term studies done is particularly difficult because trends, algorithms and habits change so quickly.
"When I started this work, TikTok wasn't even a thing … Snapchat, really has only taken off in the last decade or so," Dr Werner-Seidler said.
"It's a very fast-moving field. And so it's very, very difficult to get a handle on it before the next thing comes out."
Part of the problem is that studies have focused on overall screen time, instead of looking at what people were doing online.
"Are they FaceTiming with Grandma? Are they viewing distressing content? Are they being groomed online?" Dr Werner-Seidler said.
"This idea of nuance and it matters what people do and how they do it and how long for and with whom.
"We can't tell any of this information just by looking at how long young people spend on screens."
What social media companies know but don't say
The National Mental Health Commission has been investigating the relationship between digital tech and teen mental health.
On Friday it released its findings after months of consultation, noting the lack of longitudinal evidence and calling for further research to be made a "top priority".
Frustratingly for Dr Werner-Seidler, and other researchers in this area, the data that might solve the mystery does exist — but they can't access it.
"Big tech companies have all of this information," she said.
"If they were to share it with academics and scientists, we would be able to learn so much more, so much more quickly."
The data that has so far emerged in other ways, courtesy of lawsuits and whistleblowers such as Frances Haugen in 2021, has been disturbing.
Ms Haugen, a former Facebook employee, revealed detailed internal research showing Instagram was harmful for teenage girls.
One slide from an in-house presentation reportedly said: "We make body image issues worse for one in three teen girls."
The peak body for Australia's technology industry — whose members include social media companies Meta, Snapchat and TikTok — has defended the sector's contribution to public research.
"DIGI's relevant members have long-standing research and community partnerships in mental health and online safety, and specific policies … informed by that work," a spokesperson said.
Depending on the platform, those policies might include parental controls, avenues to report inappropriate content and seek help, customisable settings, and age limits.
Adherence to those age limits has been mostly voluntary, and the federal government is spending $6.5 million on an age verification trial in the hopes of introducing a higher standard of proof.
Some social media companies are trying to get ahead of any future legislation.
Facebook's parent company Meta announced this week it would no longer allow Facebook users to edit their birthdate to say they're over 18 without verification — a feature that's been in place on Instagram in Australia since last year.
A window for change
The public and political mood when it comes to big tech has rarely been darker.
"I've never seen the appetite [for change] as strong as it is right now," said Alice Dawkins, executive director of Reset Tech Australia.
She says there's a window for change with the federal government currently reviewing its key legislation, the Online Safety Act.
"Our online safety laws are geared at protecting people from [one] another online … [but] there's virtually nothing that can be done about protecting people from the tech itself."
As it stands, companies are rarely obliged to share information on how their products, and not just the people using them, may cause harm.
"It's highly exceptional — think about other sectors, like food, like medicine, like toys — it's incredibly routine in those sectors to have risk assessment and risk mitigation of products," Ms Dawkins said.
"There's compounding public awareness of the problem … it's never been a more appropriate time for the government to legislate."
Dr Werner-Seidler said that for now, internal data was being used by big tech to keep users scrolling for as long as possible.
"These are commercial big companies [and] they use a whole bunch of engagement strategies to keep people coming back, and that is their goal," she said.
The conversation you need to have with your kids
Jordy eventually found the courage to tell her mum what was happening to her online.
"When it got really bad I was just like, 'Mum, I need to show you … this is what's happening.'"
After that, her parents insisted she cut back her screen time but, despite everything that had already happened, she still fought it.
"I was so mean to her … I would get so angry, I'd be like, 'Mum, it's not your life,'" she said.
But that was before Jordy noticed a big improvement in her mood and her grades.
"I'm thankful every day that my mum did what she did.
"You can't ever change the fact that your kids are going to use social media," said Jordy, although boundaries were useful in her case.
"Saying to your kids, 'What are you using social media for? Why do you have to be on social media?'
"For parents out there that are struggling, I think it's that conversation you need to have with your kids.
"As a kid, you're going to get frustrated, but it's really just parents trying to protect their kids from what's out there."
Mental health disorders among young people have soared by nearly 50 per cent in 15 years. The ABC is talking to youth, parents, and researchers about what's driving this pattern, and what can be done to turn things around.
- X (formerly Twitter)
Related Stories
'there's a radicalisation happening online': fears hardcore porn could be behind rising rates of teen sexual assaults.
Why are girls suffering so much right now? The problem is bigger than you think
Teen circadian rhythms are different to the rest of us and could be key to improving their sleep and wellbeing
- Federal Government
- Mental Health
- Mental Wellbeing
- Social Media
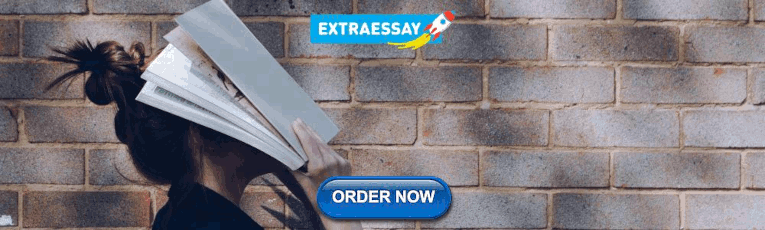
IMAGES
VIDEO
COMMENTS
Australian science has made rapid advances in the last decade in understanding eutrophication processes in inland waters and estuaries. The freshwater research on which these advances are based was triggered by well-publicised blooms of cyanobacteria during the 1980s and early 1990s, particularly a 1000 km long bloom on the Darling River. In estuaries the study which greatly enhanced our ...
Assessment of coastal eutrophication potential: a case study in the Bohai Sea. The assessment results obtained from the global eutrophication watch (Fig. 1) comparing two assessment periods, 1998 ...
Nutrient enrichment of water bodies, or eutrophication, is a growing global problem. Whereas phosphorus is the leading concern for freshwater systems, excessive nitrogen is the primary cause of eutrophication in estuaries and coastal waters ().Associated water quality impacts, including but not limited to the occurrence of harmful algal blooms (2, 3) and hypoxia (4, 5), have been widely ...
Case Study Gunaikurnai - 2 gigalitre water entitlement. Case Study Lake Hume and River Murray low dissolved oxygen event, February 2021. Case Study Pama Narrows (Yorta Yorta), also known as Barmah Choke. Case Study Almond production in Australia. In inland water, search case studies in the State of the Environment report website.
This was the case at North Lake in December 1986. This study was commenced in August 1987 to assess the problems of nuisance algal growth at North Lake and to review relevant management strategies. The results of this study showed North Lake to be highly eutrophic throughout the year.
Present address: Land & Water Australia, GPO Box 2182, 2601, Canberra, ACT, Australia (*Author for correspondence: E-mail: [email protected]) Received 19 December 2005; accepted 18 March 2005
In a study carried out by Ofir et al. (2017), a food web modeling approach have been used to predict the impact of lake biomanipulation on water quality by taking Lake Kinneret as a case study. In this study, a time dynamic model (Ecosim) has been used which is based on Ecopath model to study lake's food web (Ofir et al., 2016). The model was ...
Current scientific consensus is that inshore regions of the central and southern Great Barrier Reef, Australia, are at risk of impacts from increased nutrient (as well as sediment and pesticide) loads delivered to Reef waters. Increases in the discharge of water quality contaminants to the Reef are largely a consequence of the expansion of agricultural practices in northern Queensland ...
The known consequences of cultural eutrophication include blooms of blue-green algae (i.e., cyanobacteria, Figure 2), tainted drinking water supplies, degradation of recreational opportunities ...
Australian science has made rapid advances in the last decade in understanding eutrophication processes in inland waters and estuaries. The freshwater research on which these advances are based was triggered by well-publicised blooms of cyanobacteria during the 1980s and early 1990s, particularly a 1000 km long bloom on the Darling River. In estuaries the study which greatly enhanced our ...
A case study was conducted using Lake Erhai, and the results of the TSI methods indicated that Lake Erhai was in a mesotrophic state, and exhibited N and P co-limitation before 2006, and P limitation after 2006. (2) Lake eutrophication mechanisms were explained and specific measures for managing eutrophication were provided.
Economic impacts of eutrophication (Case study: Shellfish flavour) Some algae and diatoms impart off-flavours or bitter taints to shellfish, rendering them unpalatable and unmarketable. In 1987 in Port Phillip Bay, Melbourne, Australia, a bloom of the diatom Rhizosolenia chunii [5] [6] occurred and 3 species of shellfish within the bay, mussels ...
Numerous studies have shown that warming and eutrophication could combine to further improve the growth and metabolic rates of phytoplankton and ultimately induce the increases in the magnitude and occurrence of phytoplankton blooms (e.g., harmful algal blooms) (Winder and Sommer, 2012; Xiao et al., 2018; Lee et al., 2019). It is notable that ...
The Darling River Study ( ), the Chaffey Dam Study (Sherman et al., 2001) and the Johnstone Study ) have been the major site-specific freshwater manage- ment studies conducted in recent years (Fig ...
Eutrophication means nutrient enrichment. It causes excess primary productivity (algal overgrowth) in waterways, resulting in poor water quality and reduced amenity, and in the worst cases, algal blooms, low oxygen and fish deaths. Eutrophication can occur naturally but is a widespread problem as a result of human activities.
The results of a case study demonstrate that both methods provided similar results which corresponded with the lake's actual trophic state. Occurrence of water eutrophication The investigation from the UNEP (United Nation Environmental Protection) indicates that about 30%~40% of the lakes and reservoirs have been affected more or less by ...
The effects of eutrophication on Australia's rivers have been increasing since 1991 when more than 1,000 kilometres (km) of the Baaka/Barka - Darling River were affected by an algal bloom that killed hundreds of sheep and cattle, and caused human health impacts. ... Case Study Lake Hume and River Murray low dissolved oxygen event, February ...
Aligarh Muslim University, Aligarh 202002, India. Abstract. On the hydrological map of the world eutrophication has become the primary water quality. issue. The excessive enrichment of waters with ...
Hongliang Xu, Ying Zhang, Xiuzhen Zhu, Mingfeng Zheng; Effects of rainfall-runoff pollution on eutrophication in coastal zone: a case study in Shenzhen Bay, southern China. Hydrology Research 1 August 2019; 50 (4): 1062-1075 ... Narva Bay, Estonia (Lessin & Raudsepp 2007), the Perth coastal margin, south-western Australia (Machado ...
This study also explores the main nutrients (nitrogen and phosphorus) driving transformations in the water body and sediment. Lake Erhai was used as a case study, and it was found to be in a mesotrophic state, with N and P co-limitation before 2006, and only P limitation since 2006.
A Case Study of Lake Erie Lake Erie is the world's eleventh-largest lake in terms of surface area and is situated on the international boundary between Canada and the United States. It " supplies drinking water to 11 million [people, contains] 50% of the fish found in all of the Great Lakes combined ," and is home to numerous aquatic species.
A key finding from the study is that 'meat and meat products' contributed to almost 50% of all GHGe attributable to product purchases consumed at home in Australia in 2019, despite accounting ...
Eutrophication | Australia state of the environment 2021. We recognise Aboriginal and Torres Strait Islanders as the sovereign Traditional Owners of Australia and thank them for their stewardship of this Country, its lands, waters and skies. We respectfully acknowledge their culture and customary practices, and pay respect to their Ancestors ...
The paper "Governance Challenges on Eutrophication in Australia" is a good example of a case study on management. The term governance should be differentiated from the government in that; we do not look at the area of the government alone. This alludes to the fact that we not only look at the activities of the government itself…
The rise of social media and smartphones has coincided with an accelerating decline in teenagers' mental health — and researchers are trying to figure out whether the technology is to blame.