- Skip to primary navigation
- Skip to main content
- Skip to primary sidebar
Dream Studies Portal
Dream research, lucid dreaming, and consciousness studies
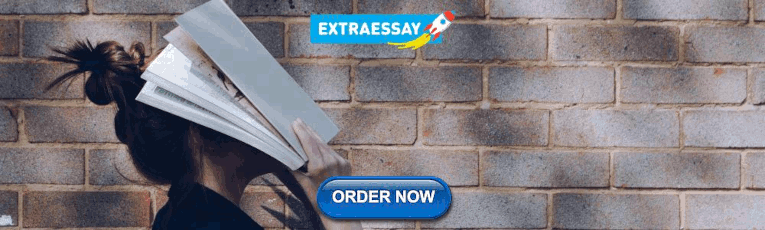
Calvin Hall and the Cognitive Theory of Dreaming
By Ryan Hurd
Any survey of modern dream research must include Calvin Hall (1909-1985). Hall was a behavioral psychologist who explored the cognitive dimensions of dreaming. His work began before the discovery of REM sleep, so little was known about the biology of sleep and dreams. Hall drew worldwide attention for his cognitive theory of dreaming , which was among the first scientific theories of dream interpretation based on quantitative analysis… rather than wishful thinking.
Dreams Images are the Embodiment of Thought
Central to Hall’s cognitive theory is that dreams are thoughts displayed in the mind’s private theater as visual concepts. Like Jung, Hall dismissed the Freudian notion that dreams are trying to cover something up. In his classic work The Meaning of Dreams (1966), Hall writes, “The images of a dream are the concrete embodiments of the dreamer’s thoughts; these images give visual expression to that which is invisible, namely, conceptions.” (p. 95).
So dreams reveal the structure of how we envision our lives, a display that is clearly valuable for anyone who remembers and studies their own dreams.
The Way We See the World
After studying thousands of dreams collected from his students and from around the world, Hall suggested that the main cognitive structures that dreams reveal include:
- conceptions of self (how we appear to ourselves, the roles we play in life)
- conceptions of others (the people in our lives and how we react to their needs
- conceptions of the world (our environment: is it a barren wasteland or a nurturing place?)
- conceptions of penalties (how we view the Man. What is allowed? What is forbidden?)
- conceptions of conflict (our inner discord and how we struggle with resolving it).
As a behavioral psychologist, Hall believed these conceptions are antecedents to our behavior in the waking world. They’re like maps to our actions, and “with these maps we are able to follow the course of man’s behavior, to understand why he selects one road rather than another, to anticipate the difficulties and obstacles he will encounter, and to predict his destinations.” (as qtd in Van De Castle, p. 190)
Content Analysis: the Hall-Van de Castle Scale
Hall’s work is still widely cited today, but his greatest legacy is the system of dream content analysis he developed with psychologist Robert Van De Castle in the 1960s.
Known as the Hall Van De Castle scale, this quantitative system scores a dream report with 16 empirical scales. Some scales are settings, objects, people, animals, and mythological creatures. You know, the sort of things you see walking down the street on any given day. (If you haven’t seen any chimeras or griffins recently, then you’re working too much). Other scales include emotions, sexual content, aggression, etc. .
The value of the project is that there are now hundreds of thousands of dreams measured using the HVdC system, creating a “baseline” for normal dreaming cognition. So researchers can add dreams from special interest groups (children, Vietnam vets, Armenian students) to measure their profiles against the norm. (see Figure 1 for an example of the possibilities)
This innovation is a huge milestone in the scientific study of dreams. Now researchers can easily get a snapshot of dreaming cognition that is measurable, quantitative, and statistically significant. Besides psychologists, this scale is still used widely today by sociologists and anthropologists.
And thanks to Hall’s student Bill Domhoff, now a powerful dream research figure in his own right, much of Hall and Van De Castle’s database is available online .
Dream content has coherent meaning—that is the main message behind Hall’s work with dreams. This view later came under fire by the controversial work of neuroscientist Allan Hobson , who implied that dreams may be nothing more than images stitched together from random brain pulses. This rift may be the central conflict in dream studies today.
Reader Interactions
December 4, 2009 at 10:16 pm
Thanks for the thorough presentation of Hall’s theory, Ryan. I’ve never encountered his name before. I’m a little bit perplexed though as to why a behavioral psychologist, like Hall, got involved in psychoanalytic research. I guess there’s not much difference between the two fields at that time. Anyway, I think his system of content analysis is indeed useful in providing quantitative data for the investigation of dreams knowing that the latter is filled with abstractions and that subjects who report their dreams may find it difficult to remove subjective interpretations while trying to recall dreams from memory.
December 6, 2009 at 8:53 pm
Ryhen, you’re right about the issues with dream recall and after-the-fact revision… that is actually one of the central criticisms of Hall and Van de Castle’s system.
That’s why it’s important to understand that dream researchers don’t study dreams per say… we study dream reports. a dream is actually a memory, and memory is highly unpredictable. the critique isn’t devastating to the enterprise of dream studies, but certainly a dangerous pothole along the path.
December 8, 2009 at 4:00 pm
The story, as I heard it from Bill Domhoff, was that Hall was originally trained as a behaviorist in the 30’s and 40’s, the heyday of that psychological system. He focused on the study of anxiety in lab rats, which he measured by giving them mild electric shocks and then counting the resulting number of feces (which are apparently an accurate index of a rat’s level of fear). I don’t know why Hall shifted toward psychoanalysis and cognitive psychology, but I find it strangely appropriate that his approach to dream content analysis has its roots in counting rat turds.
December 8, 2009 at 5:48 pm
Kelly, thanks for coming by and also for the behaviorist scoop on Hall!
December 12, 2009 at 6:54 am
Great overview of an important perspective on dreamwork. I’m very opposed to a purely materialistic “random neural firings” hypothesis about dreams.
January 31, 2010 at 11:15 pm
Some nits for you Calvin Hall’s name does not have Jr. in the title Our Dreaming Mind was published in 1994 not 93 Domhoff’s book on Finding Meaning etc was published in 1996, not 97
February 1, 2010 at 1:07 pm
thanks, Bob, for stopping by! I’ll pick those nits.
February 9, 2010 at 10:30 am
Thanks for your overview on the cognitive theory of dreams. I’m writting a research paper on dreams where I’m looking at this theory and Freud’s theory and my argument is that the cognitive theory provides the most clarity in understanding our dreams. I was wondering if you know of any study a psychologist has conducted where he has concluded that a particular dream supports the cognitive dream theory,
I have looked into Domhoff’s dreambank.net website, and found one study that I think I may be able to use in my paper;however, I was just curious to see whether you’ve come across any such study
Thank you so much for your updates on your blog, I’d appreciate a response as soon as you can
Thanks! Tina
April 16, 2010 at 10:41 am
hi Tania am not sure how late this feedback but ..try looking into Deirdre Barrett work she has numerous books and articles on that part she is the chief editor of Dreaming Journal hope this helps. Star
April 16, 2010 at 10:52 am
thanks Star — and sorry Tina — another comment that got by my radar. in general the studies that support cognitive theory are by the researchers who use cognitive theory. same goes for Freudian theory, Jungian theory, etc. There is no final authority on the meaning of dreams when it comes down to individual dreams. in my opinion — and many dream workers — the final authority is YOU. what feels right? what can you learn? studying dreaming like all cognitive artifacts is a study in meaning-making, and nobody can tell you what something means, they can only lead the way…

The Neurocognitive Theory of Dreaming : The Where, How, When, What, and Why of Dreams
G. William Domhoff is Distinguished Professor Emeritus and Research Professor at the University of California, Santa Cruz.
A comprehensive neurocognitive theory of dreaming based on the theories, methodologies, and findings of cognitive neuroscience and the psychological sciences.
G. William Domhoff's neurocognitive theory of dreaming is the only theory of dreaming that makes full use of the new neuroimaging findings on all forms of spontaneous thought and shows how well they explain the results of rigorous quantitative studies of dream content. Domhoff identifies five separate issues—neural substrates, cognitive processes, the psychological meaning of dream content, evolutionarily adaptive functions, and historically invented cultural uses—and then explores how they are intertwined. He also discusses the degree to which there is symbolism in dreams, the development of dreaming in children, and the relative frequency of emotions in the dreams of children and adults.
During dreaming, the neural substrates that support waking sensory input, task-oriented thinking, and movement are relatively deactivated. Domhoff presents the conditions that have to be fulfilled before dreaming can occur spontaneously. He describes the specific cognitive processes supported by the neural substrate of dreaming and then looks at dream reports of research participants. The “why” of dreaming, he says, may be the most counterintuitive outcome of empirical dream research. Though the question is usually framed in terms of adaptation, there is no positive evidence for an adaptive theory of dreaming. Research by anthropologists, historians, and comparative religion scholars, however, suggests that dreaming has psychological and cultural uses, with the most important of these found in religious ceremonies and healing practices. Finally, he offers suggestions for how future dream studies might take advantage of new technologies, including smart phones.
- Open the Book PDF for in another window
- Permissions
- Cite Icon Cite
The Neurocognitive Theory of Dreaming : The Where, How, When, What, and Why of Dreams By: G. William Domhoff https://doi.org/10.7551/mitpress/14679.001.0001 ISBN (electronic): 9780262370882 Publisher: The MIT Press Published: 2022
Download citation file:
- Ris (Zotero)
- Reference Manager
Table of Contents
- [ Front Matter ] Doi: https://doi.org/10.7551/mitpress/14679.003.0001 Open the PDF Link PDF for [ Front Matter ] in another window
- Introduction: Establishing a Context, Providing an Overview Doi: https://doi.org/10.7551/mitpress/14679.003.0002 Open the PDF Link PDF for Introduction: Establishing a Context, Providing an Overview in another window
- 1: Definitions, Distinctions, and Limitations Doi: https://doi.org/10.7551/mitpress/14679.003.0003 Open the PDF Link PDF for 1: Definitions, Distinctions, and Limitations in another window
- 2: Neural Substrates, Embodied Simulation, and Cognitive Insufficiencies Doi: https://doi.org/10.7551/mitpress/14679.003.0004 Open the PDF Link PDF for 2: Neural Substrates, Embodied Simulation, and Cognitive Insufficiencies in another window
- 3: Dream Content as Revealed by Quantitative Content Analysis Doi: https://doi.org/10.7551/mitpress/14679.003.0005 Open the PDF Link PDF for 3: Dream Content as Revealed by Quantitative Content Analysis in another window
- 4: Findings on Dream Content in Individual Dream Series Doi: https://doi.org/10.7551/mitpress/14679.003.0006 Open the PDF Link PDF for 4: Findings on Dream Content in Individual Dream Series in another window
- 5: The Search for Symbolism in Dreams Doi: https://doi.org/10.7551/mitpress/14679.003.0007 Open the PDF Link PDF for 5: The Search for Symbolism in Dreams in another window
- 6: The Development of Dreaming in Children Doi: https://doi.org/10.7551/mitpress/14679.003.0008 Open the PDF Link PDF for 6: The Development of Dreaming in Children in another window
- 7: The Dream Reports of Preadolescents and Adolescents Doi: https://doi.org/10.7551/mitpress/14679.003.0009 Open the PDF Link PDF for 7: The Dream Reports of Preadolescents and Adolescents in another window
- 8: The Occurrence of Emotions in Dreams Doi: https://doi.org/10.7551/mitpress/14679.003.0010 Open the PDF Link PDF for 8: The Occurrence of Emotions in Dreams in another window
- 9: An Assessment of Two Comprehensive Traditional Dream Theories Doi: https://doi.org/10.7551/mitpress/14679.003.0011 Open the PDF Link PDF for 9: An Assessment of Two Comprehensive Traditional Dream Theories in another window
- 10: Dreaming, Adaptive Functions, and Cultural Uses Doi: https://doi.org/10.7551/mitpress/14679.003.0012 Open the PDF Link PDF for 10: Dreaming, Adaptive Functions, and Cultural Uses in another window
- 11: The Neurocognitive Theory Compared to Other Dream Theories Doi: https://doi.org/10.7551/mitpress/14679.003.0013 Open the PDF Link PDF for 11: The Neurocognitive Theory Compared to Other Dream Theories in another window
- Acknowledgments Doi: https://doi.org/10.7551/mitpress/14679.003.0014 Open the PDF Link PDF for Acknowledgments in another window
- References Doi: https://doi.org/10.7551/mitpress/14679.003.0015 Open the PDF Link PDF for References in another window
- Index Doi: https://doi.org/10.7551/mitpress/14679.003.0016 Open the PDF Link PDF for Index in another window
- Open Access

A product of The MIT Press
Mit press direct.
- About MIT Press Direct
Information
- Accessibility
- For Authors
- For Customers
- For Librarians
- Direct to Open
- Media Inquiries
- Rights and Permissions
- For Advertisers
- About the MIT Press
- The MIT Press Reader
- MIT Press Blog
- Seasonal Catalogs
- MIT Press Home
- Give to the MIT Press
- Direct Service Desk
- Terms of Use
- Privacy Statement
- Crossref Member
- COUNTER Member
- The MIT Press colophon is registered in the U.S. Patent and Trademark Office
This Feature Is Available To Subscribers Only
Sign In or Create an Account
Dreams and creative problem-solving
Affiliation.
- 1 Department of Psychiatry, Harvard Medical School, Cambridge, Massachusetts.
- PMID: 28640937
- DOI: 10.1111/nyas.13412
Dreams have produced art, music, novels, films, mathematical proofs, designs for architecture, telescopes, and computers. Dreaming is essentially our brain thinking in another neurophysiologic state-and therefore it is likely to solve some problems on which our waking minds have become stuck. This neurophysiologic state is characterized by high activity in brain areas associated with imagery, so problems requiring vivid visualization are also more likely to get help from dreaming. This article reviews great historical dreams and modern laboratory research to suggest how dreams can aid creativity and problem-solving.
Keywords: REM sleep; creativity; dream incubation; dreams; problem-solving.
© 2017 New York Academy of Sciences.
Publication types
- Brain / physiology*
- Creativity*
- Dreams / physiology*
- Dreams / psychology*
- Problem Solving / physiology*

An official website of the United States government
The .gov means it’s official. Federal government websites often end in .gov or .mil. Before sharing sensitive information, make sure you’re on a federal government site.
The site is secure. The https:// ensures that you are connecting to the official website and that any information you provide is encrypted and transmitted securely.
- Publications
- Account settings
Preview improvements coming to the PMC website in October 2024. Learn More or Try it out now .
- Advanced Search
- Journal List
- HHS Author Manuscripts

The cognitive neuroscience of lucid dreaming
Benjamin baird.
a Wisconsin Institute for Sleep and Consciousness, University of Wisconsin-Madison, Madison, Wisconsin, USA
Sergio A. Mota-Rolim
b Brain Institute, Physiology Department and Onofre Lopes University Hospital - Federal University of Rio Grande do Norte, Natal, Brazil
Martin Dresler
c Donders Institute for Brain, Cognition and Behaviour, Radboud University Medical Centre, Nijmegen, The Netherlands
Lucid dreaming refers to the phenomenon of becoming aware of the fact that one is dreaming during ongoing sleep. Despite having been physiologically validated for decades, the neurobiology of lucid dreaming is still incompletely characterized. Here we review the neuroscientific literature on lucid dreaming, including electroencephalographic, neuroimaging, brain lesion, pharmacological and brain stimulation studies. Electroencephalographic studies of lucid dreaming are mostly underpowered and show mixed results. Neuroimaging data is scant but preliminary results suggest that prefrontal and parietal regions are involved in lucid dreaming. A focus of research is also to develop methods to induce lucid dreams. Combining training in mental set with cholinergic stimulation has shown promising results, while it remains unclear whether electrical brain stimulation could be used to induce lucid dreams. Finally, we discuss strategies to measure lucid dreaming, including best-practice procedures for the sleep laboratory. Lucid dreaming has clinical and scientific applications, and shows emerging potential as a methodology in the cognitive neuroscience of consciousness. Further research with larger sample sizes and refined methodology is needed.
1. Introduction
Becoming aware that one is dreaming while dreaming, what is today referred to as lucid dreaming , has been known about since antiquity. In Western literature, it may have first been mentioned by Aristotle in the fourth century BCE in the treatise On dreams of his Parva Naturali , in which he states: “often when one is asleep, there is something in consciousness which declares that what then presents itself is but a dream” ( Aristotle, 1941 , p. 624). Likewise, in Eastern cultures, particularly of the south Asian subcontinent, reports of individuals engaging in practices to cultivate awareness of dream and sleep states date back millennia ( LaBerge, 2003 ; Norbu and Katz, 1992 ; Wallace and Hodel, 2012 ). These include meditative practices specifically designed to “apprehend the dream state” ( Padmasambhava, 1998 , p. 156).
Although numerous references to lucid dreaming can be found throughout world literature (see LaBerge, 1988a for an overview), the modem nomenclature of lucid dream was not introduced until 1913 by the Dutch psychiatrist Frederik Van Eeden. In a detailed and engaging account of his personal experiences with dreams, Van Eeden (1913) referred to lucid dreams as dreams in which “…the reintegration of the psychic functions is so complete that the sleeper remembers day-life and his own condition, reaches a state of perfect awareness, and is able to direct his attention, and to attempt different acts of free volition” (pp. 149-150). Research over the last four decades has largely confirmed Van Eeden’s accounts: as we review below, evidence suggests that during lucid dreams individuals can be physiologically asleep while at the same time aware that they are dreaming, able to intentionally perform diverse actions, and in some cases remember their waking life ( Dresler et al., 2011 ; LaBerge, 1985 , 1990 ; LaBerge, 2015 ; LaBerge, Nagel, Dement and Zarcone, 1981c ; Windt, 2015 ).
Despite the fact that such personal accounts of lucid dreams have been described for centuries, the topic faced skepticism from some scientists and philosophers (e.g., Malcolm, 1959 ), in part due to the lack of objective evidence for the phenomenon. This began to change in the late 1970s and early 1980s, however, with the first validation of lucid dreaming as an objectively verifiable phenomenon occurring during rapid eye movement (REM) sleep. Building on prior research that showed that shifts in the direction of gaze within a dream can be accompanied by corresponding movements of the sleeper’s eyes ( Dement and Wolpert, 1958 ), lucid dreamers were asked to move their eyes in a distinct pre-agreed upon sequence (full-scale up-down or left-right movements) as soon as they became lucid ( Hearne, 1978 ; LaBerge et al., 1981c ).
Through this technique, which has since become the gold standard, reports of lucid dreams could be objectively verified by the presence of distinct volitional eye movement patterns as recorded in the electrooculogram (EOG) during polysomnography-verified sleep ( Figure 1 ). The most common version of the eye signaling technique asks participants to signal when they realize they are dreaming by rapidly looking all the way to the left then all the way to the right two times consecutively then back to center in the dream without pausing (referred to as left-right-left-right eye signals, abbreviated as LRLR). As can be seen in Figure 1 , the LRLR signal is readily discernable in the horizontal EOG, which exhibits a distinctive shape containing four consecutive full-scale eye movements that have larger amplitude compared to typical REMs. As we describe in detail below, lucid dreams can be validated by this method through the convergence between reports obtained after awakening of becoming lucid and making the eye movement signals during the dream, accompanied by the objective eye movement signals recorded in the EOG with concurrent polysomonographic evidence of REM sleep.

Exemplary left-right-left-right-center (LRLR) eye movement signal during polysomnographcally-verified REM sleep. Participants signal when they realize they are dreaming by rapidly looking all the way to the left (as if looking at their ear) then all the way to the right two times consecutively then back to center without pausing. The LRLR signal is readily discernable in the HEOG, which exhibits a distinctive shape of four consecutive full-scale eye movements of higher amplitude compared to typical REMs. Note high-frequency electroencephalogram (EEG) with theta rhythm (~5 Hz) and lack of alpha at OZ as well as minimal electromyogram (EMG) amplitude due to muscle atonia characteristic of REM sleep (left) compared to wakefulness (right).
The eye signaling technique also offers a way of objectively contrasting lucid REM sleep to baseline non-lucid REM sleep, providing a method to investigate the changes in brain activity associated with lucid dreaming. Furthermore, lucid dreamers can not only signal to indicate that they are aware that they are dreaming, but they can also make the eye movement signals to time-stamp the start and end of experimental tasks performed during lucid dreams ( LaBerge, 1990 ). By providing objective temporal markers, this technique has opened up a new method for studying the psychophysiology of REM sleep, allowing, for example, investigations into the neural correlates of dreamed behaviors (e.g., Dresler et al., 2011 ; Erlacher, Schredl and LaBerge, 2003 ; LaBerge, 1990 ; Oudiette et al., 2018 ). Lucid dreaming thus provides a way to establish precise psychophysiological correlations between the contents of consciousness during sleep and physiological measures, as well as enables experimental control over the content of dreams, and therefore provides a potentially highly useful experimental methodology.
While neuroscientific studies on lucid dreaming have been performed since the late 1970s, the topic has received increasing attention in recent years due to its relevance to the emerging neuroscience of consciousness. In this article, we review the existing literature on the neuroscience of lucid dreaming, including electrophysiological, neuroimaging, brain lesion, pharmacological and brain stimulation studies. Additionally, we review recent studies that illustrate how lucid dreaming can be used as a methodology in the cognitive neuroscience of consciousness. Finally, we present strategies to measure lucid dreaming both physiologically and with questionnaires, and discuss procedures to investigate lucid dreaming in the sleep laboratory.
2. Electrophysiology of lucid dreaming
As noted in the introduction, the first physiological studies of lucid dreaming began in the late 1970s and early 1980s. These pioneer works established that lucid dreams occur in REM sleep, characterized by all of the EEG features of REM sleep according to the Rechtschaffen and Kales (1968) sleep scoring criteria ( Hearne, 1978 ; LaBerge et al., 1981c ). LaBerge (1980b) also observed that lucid dreams are associated with increased physiological activation, as measured by increased phasic activity (e.g., increased REM density). Autonomic nervous system arousal (e.g., heart rate, respiration rate, and skin potential) were also found to be elevated during lucid REM sleep compared to non-lucid REM sleep ( LaBerge, Levitan and Dement, 1986 ). Additionally, lucid dreams were found to occur in REM sleep periods later in the night ( LaBerge et al., 1986 ). These findings suggest that lucid dreaming is associated with increased cortical activation ( LaBerge, Nagel, Taylor, Dement and Zarcone, 1981a ), which reaches its peak during phasic REM sleep. In addition to physiological markers of phasic activity, lucid REM sleep was found to be associated with h-reflex suppression ( Brylowski, Levitan and LaBerge, 1989 ), a spinal reflex that is reliably suppressed during REM sleep ( Hodes and Dement, 1964 ). Together these results indicate that lucid dreams occur in activated periods of REM sleep, as opposed to, for example, a state that is intermediate between waking and REM sleep.
These findings also raise the further question of whether lucid REM sleep is associated with localized activation of specific brain regions or changes in specific frequencies of neural oscillations compared to non-lucid REM sleep. In this section, we will review EEG studies that have attempted to address this question. As will be discussed below, while these studies represent important first steps toward measuring the electrophysiological changes associated with lucid dreaming, all of them have interpretive issues and most suffer from low statistical power. As a result, there is considerable discrepancy among findings. Below we group and discuss results based on the regional EEG band power changes reported to be associated with lucid REM sleep dreaming.
2.1. EEG studies of lucid REM sleep dreaming
2.1.1. central and posterior alpha.
Ogilvie and colleagues conducted some of the first studies to examine EEG spectral changes during lucid REM sleep. In an early case study, Ogilvie, Hunt, Sawicki and McGowan (1978) compared two lucid REM sleep epochs to six non-lucid REM sleep epochs and found an increase in the percentage of alpha band (8-12 Hz) power in a single central EEG channel. A follow-up study examined a larger group of ten participants for two nights in the sleep laboratory ( Ogilvie, Hunt, Tyson, Lucescu and Jeakins, 1982 ). Participants were awakened during the two periods of highest alpha power and two periods of lowest alpha power from a central EEG electrode during REM sleep, after which they were asked several questions about their lucidity, “prelucidity” (i.e., thoughts pertaining to dreaming without becoming lucid), and degree of dream control. Unfortunately, statistics for the number of lucid dreams reported by participants were not documented in this study, nor whether there was a significant difference between conditions in the number of lucid dreams. Instead, a composite measure was constructed by collapsing across pre-lucidity, lucidity and control, which was significantly elevated in the high alpha trials. Therefore, the number (if any) of lucid dreams that were captured by this procedure is unknown (see LaBerge (1988b) for further discussion).
Subsequent research has not supported the hypothesis that lucid REM sleep is associated with increased alpha activity. For example, in a follow-up study Tyson, Ogilvie and Hunt (1984) found that only pre-lucid but not lucid dreams significantly differed in alpha activity compared to non-lucid REM sleep. In another study of eight frequent lucid dreamers using a similar experimental design, Ogilvie, Hunt, Kushniruk and Newman (1983) observed no difference in the number of lucid dreams following awakenings from periods of high or low alpha activity. Furthermore, in a replication attempt of the original case report that observed an increase in alpha during lucid REM sleep ( Ogilvie et al., 1978 ), LaBerge and colleagues found no significant differences in alpha power at the same central channel (C3) from a single subject ( LaBerge, 1988b ). Finally, a later follow-up case study analyzed EEG spectral power in five lucid REM sleep epochs compared to non-lucid REM sleep periods, and observed no differences in alpha power ( Ogilvie, Vieira and Small, 1991 ). Together this evidence does not support a reliable association between alpha power and lucid dreaming. However, the limited spatial coverage of EEG montages in these studies (in several cases consisting of only one EEG channel) makes it unclear to what extent these results can be generalized to other brain areas.
2.1.2. Parietal beta
Holzinger, LaBerge and Levitan (2006) examined EEG spectral changes during lucid REM sleep in a group of eleven participants who reported prior experience with lucid dreaming. Six out of the eleven participants succeeded in becoming lucid in the sleep laboratory, and some during multiple REM periods, for a total of 16 signal-verified lucid dreams. The authors found increased power in the low beta frequency range (13-19 Hz) in parietal electrodes for lucid compared to non-lucid REM sleep. This study has the advantage of analyzing a larger number of lucid REM sleep periods. However, a limitation is that EEG signals were only evaluated at four electrodes (F3, F4, P3, P4). Consequently, localized changes in EEG spectra may have been missed by the low spatial resolution. Furthermore, due to technical limitations, the online low-pass filter for the EEG recordings in this study was set at 35 Hz, and therefore changes in higher-frequency activity were unable to be evaluated.
The potential functional significance of increased low beta band power in parietal areas to lucid dreaming is not understood. Holzinger et al. (2006) speculated that the increased beta power in the parietal EEG could reflect the understanding of the meaning of the words “This is a dream.” As discussed below, recent neuroimaging studies have linked parietal regions to several other cognitive functions associated with lucid dreaming, including self-reflection ( Kjaer, Nowak and Lou, 2002 ; Lou et al., 2004 ), episodic memory ( Berryhill, Phuong, Picasso, Cabeza and Olson, 2007 ; Wagner, Shannon, Kahn and Buckner, 2005 ) and agency ( Cavanna and Trimble, 2006 ), suggesting several other possible interpretations of the results. Neural oscillations in this frequency range were originally thought to reflect sensorimotor behavior; however, evidence now suggests that oscillations in this range could play a role in cognitive processing as well as facilitate large-scale neural integration (e.g., Donner and Siegel, 2011 ; Engel and Fries, 2010 ). If these results can be replicated in future studies, one hypothesis is that the increased oscillatory activity in the beta band could reflect a mechanism of integration between parietal regions and other areas, which, in some way still to be understood, helps facilitate lucid dreaming. However, it is also possible that differences in sensorimotor behavior between lucid and non-lucid REM sleep could boost brain rhythms that overlap with this frequency range ( Koshino and Niedermeyer, 1975 ; Pfurtscheller, Stancak and Neuper, 1996 ; Vanni, Portin, Virsu and Hari, 1999 ). Additional research is needed to clarify these findings.
2.1.3. Frontolateral gamma
Mota-Rolim et al. (2008) and Voss, Holzmann, Tuin and Hobson (2009) reported increased gamma band (40 Hz) power in frontolateral scalp electrodes during lucid compared to non-lucid REM sleep in three participants each. However, besides an unsuccessful replication in patients with narcolepsy ( Dodet et al., 2014 ), interpretation of these results are restricted by several experimental limitations, including the small sample size ( LaBerge, 2010 ). Importantly, caution is warranted in interpreting these findings given that, as briefly discussed by Voss et al. (2009) , scalp measurement of cortical gamma, particularly when selectively localized in the frontal and periorbital regions, may be confounded with mircrosaccades. Ocular myogenic artifacts, which occur during both saccades and microsaccades and are distinct from the artifacts associated with corneo-retinal dipole offsets, may confound scalp measurement of gamma activity. One type of such artifacts is referred to as the saccadic spike potential (SP), which occurs due to contraction of the ocular muscles during both saccades and microsaccades ( Yuval-Greenberg and Deouell, 2009 ; Yuval-Greenberg, Tomer, Keren, Nelken and Deouell, 2008 ). The influence of the SP artifact on gamma power was overlooked for a number of years; however, the need to account for this artifact on scalp measurement of induced gamma activity has now been thoroughly documented (e.g., Hipp and Siegel, 2013 ; Keren, Yuval-Greenberg and Deouell, 2010 ).
Voss et al. (2009) corrected for eye movement artifacts using regression of the EOG signal ( Gratton, Coles and Donchin, 1983 ) and by computing current source densities (CSD) in addition to scalp potentials. However, regression-based correction procedures are insufficient to remove the SP artifact, and while the CSD derivation attenuates the SP artifact at posterior channels, it is not sufficient to remove it at anterior scalp locations ( Keren et al., 2010 ; Yuval-Greenberg and Deouell, 2009 ). As Keren et al. (2010) state: “In conclusion, SCD [CSD] seems to be effective in attenuating the SP effect at posterior sites. However at sites anterior to Cz and closer to the orbits efficacy gradually decreases, preserving the temporal and spectral signature of the SP and its amplitude relative to baseline.” (p. 2258). The influence of the SP artifact on gamma band power in the comparison of lucid to non-lucid REM sleep is particularly relevant given that, as noted above, lucid REM sleep has been associated with increased phasic activation and higher eye movement density (e.g., LaBerge, 1990 ).
It is important to note that the need to account for the SP artifact does not preclude a potential association between increased frontal gamma power and lucid REM sleep. Indeed, given the link between gamma band power, local field potentials (LFPs) and the blood-oxygen-level dependent (BOLD) signal ( Lachaux et al., 2007 ; Nir et al., 2007 ), if the transition from non-lucid to lucid REM sleep involves activation or recruitment of additional frontal brain regions (a plausible hypothesis, also in light of the findings of Dresler et al. (2012) ; see Neuroimaging of lucid dreaming below), regional increases in gamma power might be predicted. However, the spatial topography and frequency localization of any such effects are likely to be strongly influenced by the correction and removal of the SP artifact.
With the aforementioned considerations in mind, more research is needed to clarify whether the increase in frontolateral gamma power during lucid REM sleep observed by Mota-Rolim et al. (2008) and Voss et al. (2009) reflects ocular myogenic or neural activity. Furthermore, future studies evaluating the relationship between lucid REM sleep and gamma activity from sensor-level EEG, particularly at anterior electrodes, need to control for the SP artifact in order for the results to be interpretable. Several methods have been shown to be suitable for removal this artifact, including direct identification and removal of contaminated data by rejecting overlapping windows of time-frequency transformed data or data correction using independent component analysis ( Hipp and Siegel, 2013 ). A study that evaluates the effect of direct removal and/or correction of the SP artifact on gamma power during lucid contrasted with baseline REM sleep would be an important addition to the literature. In summary, studies that rigorously control for myogenic artifacts, and in particular the SP artifact, are needed before conclusions can be drawn regarding the relationship between lucid REM sleep and frontal gamma activity.
2.1.4. Fronto-central delta
Dodet, Chavez, Leu-Semenescu, Golmard and Arnulf (2014) evaluated changes in EEG band power during lucid REM sleep in a group of narcoleptic patients. Given that narcoleptic patients often report a high rate of lucid dreams ( Rak, Beitinger, Steiger, Schredl and Dresler, 2015 ), they are a potentially useful population for cognitive neuroscience studies of lucid dreaming. In the experiment, while both control and narcoleptic patients reported achieving lucidity and performing the LRLR signals during overnight and afternoon nap recordings, only the eye signals of the patients during the nap recordings could be unambiguously identified. This is likely at least partially attributable to the specific instructions used for making the eye movement signal (see Section 9 below for discussion of this issue). Despite this, the study succeeded in recording 14 signal-verified lucid dreams during naps from seven narcoleptic patients. The main finding was that EEG power was reduced in the delta band during lucid REM sleep at frontal and central electrodes. The study also reported that the coherence between several electrodes was reduced in lucid compared to non-lucid REM sleep in delta, theta, beta and gamma bands, but these differences are difficult to interpret since no statistics were reported for this analysis and no corrections for multiple comparisons were made.
A limitation of the Dodet et al. (2014) study is that EEG signals were only evaluated at six electrodes, and only in frontal and central scalp regions (Fp1, Fp2, F7, F8, C3, C4). Parietal electrodes were not included in the EEG montage, and occipital channels reportedly could not be evaluated due to noise. Thus, it is possible that local changes in EEG spectra in posterior regions, such as parietal or occipital areas, were missed due to the limited electrode montage.
The finding of lower delta activity during lucid REM sleep is in line with previous observations that lucid dreams tend to occur during periods of increased cortical activation ( LaBerge, 1990 ). Specifically, slow waves, reflected by delta (~0.5-4 Hz) power in the EEG, are associated with neuronal down states (“off’ periods) in which neurons are hyperpolarized ( Steriade, Timofeev and Grenier, 2001 ). Decreased delta power (EEG activation) therefore reflects recovery of neural activity. While the bi-stability between “on” and “ off’ periods is a central feature of non-REM sleep, slow wave activity has also been observed in REM sleep ( Baird et al., 2018b ; Funk, Honjoh, Rodriguez, Cirelli and Tononi, 2016 ). Neuronal down states have also been linked to the loss of consciousness during both anesthesia and sleep ( Purdon et al., 2013 ; Tononi and Massimini, 2008 ), which is hypothesized to be related to the breakdown of causal interactions between bi-stable neurons ( Pigorini et al., 2015 ; Tononi, Boly, Massimini and Koch, 2016 ). Therefore, one potential explanation is that this finding reflects reduced bi-stability and increased causal interactions between cortical neurons in these areas during lucid REM sleep. Notably, reduced delta power in posterior cortex has been found to be associated with dreaming as opposed to dreamless sleep in both REM and NREM sleep ( Siclari et al., 2017 ). An intriguing speculation based on these results is therefore that this reduction in delta power also extends to frontal regions during lucid REM sleep dreaming. However, it remains to be seen whether these findings can be replicated and whether the results generalize to non-clinical populations.
2.2. General discussion of EEG studies of lucid REM sleep
In summary, EEG studies show substantial disagreement regarding the spatial and spectral changes associated with lucid dreaming. As reviewed above, different studies have observed an increase in central or posterior alpha, parietal beta, frontolateral gamma or a reduction in frontocentral delta during lucid compared to baseline REM sleep. Aside from the general uncertainty in the results of some studies due to low statistical power, these discrepant results might be partially explained by the use of limited electrode montages and evaluation of different EEG frequency bands. In the spatial domain in particular, many studies have used less than six scalp electrodes, in several cases only covering some scalp regions but not others, precluding analysis of EEG activity in regions in which significant effects were found in other studies. For instance, the study by Dodet et al. (2014) did not include electrodes in parietal or occipital regions, precluding the possibility to replicate the findings of increased parietal beta by Holzinger et al. (2006) . Studies by Ogilvie et al. (1978 , 1982 ) evaluated only a single central EEG channel (C3). These non-overlapping spatial montages limit the comparison of results across some of these studies.
Another factor that might contribute to the discrepant findings is the fact that lucid dreaming can be achieved and executed in different ways. For example, the observed changes in the EEG during lucid REM sleep might depend in part on the degree of vividness, working memory, emotional tone, self-consciousness, attention and insight, which could vary across individuals as well as specific dreams. Relatedly, different subjective experiences and contents during lucid dreams plausibly have their own neurobiological substrates ( Mota-Rolim, Erlacher, Tort, Araujo and Ribeiro, 2010 ), just as in non-lucid dreams ( Siclari et al., 2017 ). Changes in brain activity during lucidity may also partly depend on how experienced the lucid dreamer is. For instance, lucid dreams of less experienced individuals may often be more ephemeral and involve less control over dream content, while more experienced lucid dreamers may be more likely to have longer and more stable lucid dreams, as well as the capacity to exert greater amounts of control. This might lead to a more distinct signal in the EEG for experienced lucid dreamers on the one hand, but also presumably less neural activity related to the effort needed to maintain the state (neural efficiency). In line with this, Dodet et al. (2014) suggested that the mental effort needed to achieve and sustain lucidity might be reduced in narcoleptic patients, who may access the lucid REM sleep state with less effort.
These comments should not be taken to indicate that there is not a consistent neurobiology of lucid dreaming. However, it does suggest that analysis of the EEG spectral changes associated with lucid dreaming used in previous studies may need to be optimized for detecting more subtle and localized effects. All studies reviewed above have measured the average power over a given spectral band and region over comparably long time intervals. However, it is possible that lucid dreaming is associated with spectral changes that can only be detected by a better time resolved analysis, such as time-frequency analysis, that may be overlooked by averaging over large windows in time or frequency space.
Furthermore, these considerations emphasize the need for more careful assessment of the phenomenology of lucid dreams. In this regard, we would like to note that it is plausible that there are at least two different neural signatures associated with lucid dreaming. The first captures what might be termed the “moment of lucidity”—that is, the transient moment of meta-awareness in which one has the metacognitive insight that one is currently dreaming ( Schooler, 2002 ). The second captures potential sustained differences in brain activity between lucid and non-lucid REM sleep dreaming. This second neural signature is unlikely to be a signature of meta-awareness per se, as during lucid dreams individuals do not continuously engage in metacognitive reflection on their state of consciousness. Rather, this second signature captures the “state-shift” in consciousness that occurs from non-lucid to lucid dreaming, with enhanced volition, episodic memory and accessibility of metacognition ( Dresler et al., 2014 ; Spoormaker, Czisch and Dresler, 2010 ). Changes in these aspects of cognition in the shift to lucidity have been hypothesized to reflect an overall change in the conscious experience of being a cognitive subject ( Windt and Metzinger, 2007 ). Both the physiological correlates of the moment of lucidity as well as overall differences in brain activity between lucid and non-lucid dreams are interesting research targets. However, these research targets are at least conceptually distinct, a point that has, in our view, not received adequate attention in the research literature. To our knowledge, no studies have yet evaluated differences in brain activity with EEG or functional magnetic resonance imaging (fMRI) specifically associated with the moment of lucidity, and this remains an interesting question for future work.
Larger sample sizes are needed in future studies to achieve adequate statistical power. One way to approach this would be to undertake more extensive population screening for high-frequency lucid dreamers. For example, through mass surveys, thousands of potential participants could be screened and the top few percent reporting the highest lucid dream frequency could be selected for training before undergoing sleep laboratory recordings. As we discuss below, new techniques for lucid dream induction also have the potential to enable efficient collection of larger datasets.
Overall, studies with higher statistical power, better assessment of phenomenological content, higher spatial resolution EEG montages, and more sophisticated analysis of the EEG signal will be needed to address these issues and shed light on the conflicting findings of EEG studies of lucid dreams conducted to date. Studies using high-density EEG would also be valuable, enabling both higher temporal as well as higher spatial resolution analysis of neural oscillatory activity. Source modeling of such data could also potentially be informative for localizing changes in neural oscillations associated with lucid dreaming to specific cortical areas, though it remains unclear whether methods for source localization will be able to produce a valid specification of the generators relevant for lucid dreaming. In particular, the generators might be distributed widely in the brain and active concurrently.
Another interesting question with respect to the electrophysiology of lucid dreaming is whether there are possible sleep pattern traits that are associated with lucid dreaming. It would be informative to investigate whether there are common sleep patterns seen in frequent lucid dreamers compared to non-frequent lucid dreamers. For example, do frequent lucid dreamers tend to have more phasic REM sleep, or more fragmented REM sleep with a higher number of transitions (especially gradual transitions) between REM sleep and waking? As far as we know, no study has investigated lucid dreaming with this approach; therefore, studies addressing these questions would be valuable.
2.3. EEG studies of lucid dreaming during non-REM sleep
The activated EEG of REM sleep was originally thought to be exclusively associated with dreaming ( Antrobus and Antrobus, 1967 ; Dement and Wolpert, 1958 ), while the low-frequency activity of non-REM (NREM) sleep was thought to be associated with the absence of dreaming. Subsequent research has shown, however, that participants report dreams or related forms of sleep mentation in up to 70% of awakenings from NREM sleep ( Siclari et al., 2017 ; Siclari, LaRocque, Postle and Tononi, 2013 ; Stickgold, Malia, Fosse and Hobson, 2001 ). NREM dreams tend to be less emotional and visually vivid, as well as more thought-like ( Cavallero, Cicogna, Natale, Occhionero and Zito, 1992 ; Hobson, Pace-Schott and Stickgold, 2000 ). Research suggests that lucid dreams, on the other hand, are predominantly a REM sleep phenomenon ( LaBerge et al., 1986 ; LaBerge et al., 1981c ). However, this does not imply that lucid dreams cannot occur during NREM sleep. There have been several reports of lucid dreams during NREM sleep stages N1 (transition from wake state to sleep) and N2 (consolidated light sleep), although in many of the published cases it is uncertain whether the lucid episode occurred in an unambiguous stage of NREM sleep ( Dane and Van de Caslte, 1984 ; LaBerge, 1980b , 1990 ; LaBerge et al., 1981c ; Stumbrys and Erlacher, 2012 ).
In one study, LaBerge (1980b) recorded polysomnography from a single subject who reported frequently experiencing lucid dreams at sleep onset. The participant rested quietly while drifting to sleep and upon falling asleep was awakened and asked for a dream report. Forty-two dream reports were collected over three nights, 25 of which the participant reported as a lucid dream, and all of which reportedly occurred during stage N1. However, the dream reports were mostly short “dreamlets”, thus it is plausible that these N1 lucid dreams could differ phenomenologically from REM sleep lucid dreams. Furthermore, none of these lucid dreams were verified with eye movement signals. In another study, two participants reported lucid dreams upon spontaneous awakening from N1 and N2 ( LaBerge et al., 1981c ). In the N2 instance, the participant reported only a brief moment of lucidity just before waking up. Furthermore, the participant did not make eye movement signals to time-stamp the moment of lucidity, and it is therefore difficult to ascertain whether the moment of lucidity occurred in the process of awakening. The N1 case was also ambiguous: while in this case the participant reported making eye movements to signal lucidity, the signals could not be verified on the polysomnogram.
In a study on the effects of posthypnotic suggestion on lucid dreaming, Dane and Van de Caslte (1984) tested hypnotically susceptible females with no prior experience in lucid dreaming. Importantly for the present discussion, lucid dreams were reported following awakening from both REM and NREM sleep. Five lucid dreams were reported in total from stage N2, but in all cases the LRLR eye signal occurred after arousal/awakening, and thus none of these could be objectively confirmed. However, several N1 lucid dreams were confirmed by LRLR signaling. This study was thus the first to provide objective evidence for lucid dreaming during stage 1 NREM sleep. However, as noted above, how these N1 dreams compare phenomenologically to REM sleep lucid dreams remains unclear.
Stumbrys and Erlacher (2012) reported two potential cases of lucid dreams during NREM with eye signaling. However, due to the study protocol, the experimenters could only collect dream reports the following morning. In the first case, the participant reported the next morning making an eye movement signal in a lucid dream early in the night, but given the long amount of time between the report and the signal there is uncertainty whether the report corresponds to the observed signal during NREM sleep. Furthermore, while the two 30-second polysomnography epochs for sleep scoring preceding the eye signal appear to be unambiguous N2 sleep, the EEG dynamic shifts during the 30-second epoch containing the eye-signal to lower amplitude activity without apparent spindles or K-complexes. Without knowing the stage of the epochs following the eye signal, which was not reported in the study, it is possible that the eye signal occurred in a transitional sleep stage. In the second case reported by Stumbrys and Erlacher (2012) , the eye signals occurred with some signs of arousal and the participant had no memory of executing the eye signals the following morning. These data therefore provide ambiguous evidence for signal-verified lucid dreams during NREM sleep.
In three further case reports of eye movement signaled NREM lucid dreams, one case was reported to occur in N1 and two cases in N2 visually scored sleep stages ( Mota-Rolim et al., 2015 ). The first case was scored as N1, since an increase in theta (4-7 Hz) and a decrease in alpha (7-14 Hz) power was observed in more than half of the 30 second scoring epoch, meeting the AASM criteria for classification of stage N1 sleep. The other two cases were scored as occurring during N2 episodes since they had spindles and K-complexes in the 30 second scoring epoch with the eye signals. These data thus replicate signal-verification of N1 lucid dreams and provide preliminary evidence for signal-verified N2 lucid dreams.
Together, these results suggest that although most lucid dreams occur during REM sleep, they can also occur during NREM sleep. However, additional studies providing objective evidence of NREM lucid dreams confirmed by eye-signaling, particularly in N2 sleep, are needed. Currently there are no reports of lucid dreams recorded during NREM stage 3 (N3), also known as deep sleep, or slow wave sleep. While there are intriguing reports of practitioners of both Transcendental Meditation and Tibetan Dream Yoga claiming to have developed the ability to maintain a type of lucid awareness throughout the entire sleep cycle, including also states of “lucid dreamless sleep” ( Gackenbach, Cranson and Alexander, 1986 ; Mason and Orme-Johnson, 2010 ; Wallace, 2013 ; Windt, Nielsen and Thompson, 2016 ), these claims have not been corroborated with physiological measures. However, several studies have found a relationship between meditation practice and lucid dreaming (e.g., Baird, Riedner, Boly, Davidson and Tononi, 2018c ; Gackenbach et al., 1986 ; Mota-Rolim et al., 2013 ; Stumbrys, Erlacher and Malinowski, 2015 ), which could be due to changes in REM sleep patterns induced by meditation practice and/or to neurocognitive changes associated with meditation practice (e.g., increased mental control or meta-awareness).
3. Neuroimaging of lucid dreaming
As noted, dream-like mental activity can be observed during all sleep stages. However, REM sleep dreams tend to be more vivid, emotional, bizarre, and more often include a narrative structure ( Cavallero et al., 1992 ; Hobson et al., 2000 ). These phenomenological characteristics have been suggested to be associated with the neural activation and deactivation patterns observed during REM sleep (e.g., Nir and Tononi, 2010 ). For example, higher visual areas show increased regional cerebral blood flow during REM sleep compared to both wakefulness and slow wave sleep ( Braun et al., 1998 ), which is in line with the visuospatial experiences that are common during REM sleep dreaming (e.g., Windt, 2010 ). Additionally, the amygdala, medial prefrontal cortex and anterior cingulate cortex show increased regional cerebral blood flow during REM sleep ( Braun et al., 1997 ; Maquet et al., 1996 ). All of these brain areas have been implicated in emotional processing, mirroring the intense emotions that can be experienced in REM sleep dreams. In contrast, the anterior prefrontal cortex (aPFC) and parietal cortex, including the inferior parietal lobule and precuneus, show low regional cerebral blood flow during normal REM sleep ( Braun et al., 1997 ; Maquet et al., 1996 ). Deactivation of these regions has been postulated to underlie the diminished insight into the global state of consciousness and restricted volitional control typical of non-lucid dreaming (e.g., Hobson and Pace-Schott, 2002 ; Nir and Tononi, 2010 ).
3.1. Neuroimaging lucid REM sleep dreaming
At the current time, there is only one fMRI study of lucid REM sleep, and it is a case study ( Dresler et al., 2012 ). Two separate signal-verified lucid dreams were recorded from a single subject during EEG-verified REM sleep inside the MRI scanner. Compared to non-lucid REM sleep, lucid REM sleep showed increased fMRI BOLD signal in a number of cortical regions, including the superior frontal gyrus, aPFC, medial and lateral parietal cortex, inferior/middle temporal gyri and occipital cortex ( Figure 2a ). Interestingly, several of these regions, particularly the parietal regions and frontal pole, are areas that, as noted above, consistently show reduced regional cerebral blood flow during non-lucid REM sleep compared to wakefulness ( Nir and Tononi, 2010 ).

a) Blood-oxygen-level dependent (BOLD) activation in fMRI case study of lucid dreaming ( Dresler et al., 2012 ). Clusters show regions with significantly increased BOLD signal during lucid REM sleep ( p FDR < 0.005) in the left lateral hemisphere view (left) and right lateral hemisphere view (right). Increased activity was observed in anterior prefrontal cortex (aPFC), medial and lateral parietal cortex, including the supramarginal and angular gyrus and inferior/middle temporal gyrus during lucid REM sleep contrasted with non-lucid REM sleep. b) Seed-based resting-state functional connectivity differences between frequent lucid dreamers and controls ( Baird et al., 2018a ). To estimate connectivity, spherical regions-of-interest were defined in aPFC based on the peak voxel reported in Dresler et al. (2012) (red circle). Frequent lucid dreamers had increased resting-state functional connectivity between left aPFC and bilateral angular gyrus, bilateral middle temportal gyrus and right inferior frontal gyrus. All clusters are significant at p <0.05, corrected for multiple comparisons at the cluster level.
Evidence linking frontopolar cortex to lucid dreaming is consistent with a role of this region in metacognition and self-reflection. For example, research has found that aPFC shows increased activation during self-reflection on internal states, such as the evaluation of one’s own thoughts and feelings ( Christoff, Ream, Geddes and Gabrieli, 2003 ; McCaig, Dixon, Keramatian, Liu and Christoff, 2011 ). Individuals can also learn to voluntarily modulate activity in aPFC through a metacognitive awareness strategy ( McCaig et al., 2011 ). Furthermore, inter-individual variance in metacognitive ability has also been linked to aPFC gray matter volume ( Fleming, Weil, Nagy, Dolan and Rees, 2010 ) and aPFC resting-state functional connectivity ( Baird, Smallwood, Gorgolewski and Margulies, 2013 ). Finally, patients with damage to this region frequently display metacognitive deficits such as inability to monitor disease symptoms or accurately appraise their cognitive functioning ( Joseph, 1999 ; Schmitz, Rowley, Kawahara and Johnson, 2006 ), which might be compared to the lack of metacognitive insight into the state of consciousness characteristic of non-lucid REM sleep dreams ( Dresler et al., 2015 ).
Dresler et al. (2012) additionally observed increased BOLD signal during lucid dreaming in the bilateral precuneus and inferior parietal lobules (angular and supramarginal gyri). As noted above, parietal cortex and the precuneus in particular has been implicated in self-referential processing, episodic memory, and the experience of agency ( Cavanna and Trimble, 2006 ), mirroring the increase of these cognitive capabilities during lucid dreaming. Finally, activation increases during lucid dreaming were also found in some occipital and inferior temporal regions, which are part of the ventral stream of visual processing involved in conscious visual perception ( Rees et al., 2002 ). While these activations may seem puzzling at first sight, as non-lucid dreams are also characterized by vivid dream imagery, they are in line with reports that lucid dreams can be associated with increased visual vividness and clarity of the dream scene (e.g., Green, 1968 ).
There are several limitations to the study by Dresler et al. (2012) that are important to note. First, as mentioned above, the findings are based on observations from a single subject and caution is therefore warranted in generalizing from the results. Currently no group-level fMRI study of lucid dreaming has been conducted, and such a study, along with systematic replications, will be needed before firm conclusions can be drawn. Another limitation is that, as described below (see Section 7.1 ), the participant was performing a task during the lucid REM sleep segment (repeated eye signaling and hand clenching). While Dresler and colleagues accounted for task activation via nuisance regression of the left and right fist clenching task, it is possible that some of the activations observed still partially reflect task execution and maintenance of attention/task-set rather than lucidity per se. One way to address this in future studies would be to contrast periods of lucid REM sleep when the participant is not performing an explicit task to non-lucid REM sleep, i.e., a “no-task, within-state paradigm” ( Siclari et al., 2013 ).
3.2. Neuroimaging individual differences in lucid dreaming
Several studies have taken an individual differences approach to neuroimaging of lucid dreaming. While most people spontaneously experience lucid dreams infrequently, there is substantial variation in lucid dream frequency, ranging, by current estimates, from never (approximately 40-50%) to monthly (approximately 20%) to a small percentage of people that report lucid dreams several times per week or in some cases every night ( Mota-Rolim et al., 2013 ; Saunders, Roe, Smith and Clegg, 2016 ; Snyder and Gackenbach, 1988 ). This variation invites the question of whether individuals who experience frequent lucid dreams show differences in anatomical or functional properties of the brain. Studies addressing this question can provide complementary evidence on the neurobiology of lucid dreaming.
In the first neuroimaging study to evaluate the relationship between lucid dream frequency and brain anatomy, Filevich, Dresler, Brick and Kuhn (2015) measured whole-brain gray matter volume using voxel-based morphometry in individuals with higher (“high lucidity”) vs. lower (“low lucidity”) scores on a scale assessing the frequency of lucid dreams and/or dream content hypothesized to be related to lucidity. Consistent with the hypothesized connection between the metacognitive functions of aPFC and lucid dreaming discussed above, the study found increased gray matter volume in two regions of the frontal pole (BA9/10), as well as the right anterior cingulate cortex, left supplementary motor area and bilateral hippocampus in the high lucidity group. Additionally, the two identified frontopolar regions showed higher BOLD signal in the monitoring component of a metacognitive thought-monitoring task performed while awake.
A limitation of the study by Filevich et al. (2015) is that participants were not frequent lucid dreamers per se, but rather subjects from a database who scored higher relative to other participants on the scale. Lucid dream frequency for the two groups was not reported in the study, thus it remains unclear to what extent the “high lucidity” group experienced frequent lucid dreams in absolute terms. Furthermore, the composite measure of dreaming used to distinguish the two groups measured not only frequency of lucid dreams but also different dimensions of dream content. While several of these content dimensions have been found to be higher in lucid dreams ( Voss, Schermelleh-Engel, Windt, Frenzel and Hobson, 2013 ), it is likely that several of these dimensions also co-vary more generally with dream recall and/or cognitive content in dreams unrelated to lucidity. As a consequence, as the authors note, some of the results could have been partly influenced by differences in dreaming “styles”, content or dream recall.
More recently, Baird, Castelnovo, Gosseries and Tononi (2018a) evaluated a sample of high-frequency lucid dreamers who reported lucid dreams in the range of three to four times per week to multiple times per night compared to a control group who reported lucid dreams once per year or less. The frequent lucid dream group and control group were case-control matched on age, gender, and dream recall frequency. Based on the previous research reviewed above, the primary aim of the study was to investigate whether individuals who have frequent lucid dreams would show increased gray matter density and/or resting-state functional connectivity of aPFC. Consistent with this, compared to the control group, individuals who reported frequent lucid dreams showed increased resting-state functional connectivity between the left aPFC and the bilateral angular gyrus, bilateral middle temporal gyrus and right inferior frontal gyrus ( Figure 2b ). The frequent lucid dream group also showed decreased functional connectivity between left aPFC and bilateral insula. Whole-brain graph-theoretic analysis revealed that left aPFC had increased node degree and strength in the frequent lucid dream group compared to the control group. However, in contrast to the findings of Filevich et al. (2015) , no significant differences in gray matter density were observed between groups in either a whole-brain analysis or an aPFC region-of-interest analysis.
Given the link to metacognition, it has also been suggested that lucid dreaming may be linked to large-scale networks that regulate executive control processes, in particular the frontoparietal control network ( Dresler et al., 2015 ; Spoormaker et al., 2010 ). To address this question, Baird et al. (2018a) additionally evaluated the association between frequent lucid dreaming and connectivity within established large-scale brain networks, including the frontoparietal control network. Consistent with a link between the frontoparietal control network and lucid dreaming, Baird and colleagues found that frequent lucid dreamers had increased functional connectivity between aPFC and a network of regions that showed the greatest overlap with a frontoparietal control sub-network ( Dixon et al., 2018 ; Yeo et al., 2011 ). However, neither connectivity within the frontoparietal control network broadly defined through meta-analysis (or within or between any other large-scale networks), nor connectivity within frontoparietal control sub-networks, as defined through parcellation of resting-state networks, showed significant differences between the lucid dream group and the control group. The authors speculate that this could be attributed to both the partial overlap of the regions that showed increased aPFC connectivity in lucid dreamers with the frontoparietal control network. However, it is important to keep in mind that while this study did not find a significant difference in resting-state connectivity within the frontoparietal network in frequent lucid dreamers, it remains an open question whether lucid REM sleep dreams show increased connectivity within the frontoparietal control network compared to non-lucid REM sleep dreams.
Overall, the resting-state connectivity results of Baird et al. (2018a) converge with the fMRI case study of lucid REM sleep dreaming described above ( Dresler et al., 2012 ), which found that an overlapping network of brain areas increased fMRI BOLD signal during lucid compared to baseline REM sleep, including bilateral aPFC, bilateral inferior parietal lobule (including the angular gyrus), and bilateral middle temporal gyrus ( Figure 2 ). Together, these results suggest that increased intrinsic functional connectivity between aPFC and the angular gyrus/middle temporal gyrus—regions that, as reviewed above, show reduced activity in REM sleep ( Nir and Tononi, 2010 ) and increased activity during lucid REM sleep ( Dresler et al., 2012 )—is associated with the tendency to have frequent lucid dreams. However, while the convergence between these two preliminary studies is encouraging, the paucity of neuroimaging data on this question limits strong conclusions at the current time.
3.3. Future directions for neuroimaging of lucid dreaming
Neuroimaging studies of lucid REM sleep using larger samples sizes are needed. In particular, a group-level fMRI study of lucid REM sleep dreaming using a no-task, within-state paradigm is perhaps the most important next step in this line of research. In addition to evaluating activation and deactivation as revealed by changes in BOLD signal, it would also be informative to evaluate differences in functional connectivity in such a study. For example, as noted, one question that arises from the above neuroimaging findings is whether there could be increased connectivity within the network of regions identified in Dresler et al. (2012) and Baird et al. (2018a) during lucid contrasted with non-lucid REM sleep dreaming. Furthermore, building on the individual differences results, in future work it would also be interesting to evaluate whether high frequency lucid dreamers show increased functional connectivity and/or higher BOLD signal in these brain areas during baseline REM sleep. If so, this could suggest that it may be possible to bias the brain toward increased metacognitive awareness of dreaming during REM sleep, for example, as discussed in the next sections on induction of lucid dreams, through techniques to increase activation of these regions.
In line with these remarks, it has been suggested that not only regional activation of frontoparietal brain areas but also connectivity between these regions could be important for lucidity to emerge during REM sleep ( Spoormaker et al., 2010 ). Indeed, while activation in these frontal and parietal regions has been linked to key functions associated with lucid dreaming, including metacognition, as discussed above, regional activations and metabolic increases in these regions have also been observed during states of global unconsciousness and subliminal information processing. For instance, subliminally presented no-go stimuli during a response inhibition task activate frontoparietal cortices in the absence of awareness ( van Gaal, Ridderinkhof, Scholte and Lamme, 2010 ). Moreover, loss of consciousness during the tonic phase of generalized tonic-clonic seizures is associated with a transient increase rather than decrease in metabolism in frontoparietal cortex ( Blumenfeld, 2008 ; Engel, Kuhl and Phelps, 1982 ).
Recent findings have suggested that a potentially more sensitive marker of unconscious states may be reduced connectivity between frontoparietal areas, particularly from frontal to parietal regions. For example, several neuroimaging studies of patients in a persistent vegetative state or under different categories of general anesthetics have shown a specific impairment of the backward connectivity from frontal to parietal regions ( Boly et al., 2011 ; Boly et al., 2012 ; Lee et al., 2013 ). These findings converge with theoretical work and computational modeling ( Tononi, 2011 ), which has suggested a link between consciousness and effective connectivity within a neural architecture, or the capacity of a set of neural elements to exert causal influence over other neural groups in a system. At present, it is unclear whether this reduction of top-down frontoparietal connectivity is linked to changes in global brain activity, alterations in primary consciousness (i.e., subjective, phenomenal states of seeing, hearing or feeling), or whether it could relate to self-awareness (i.e., explicit conscious awareness of oneself and one’s state). A common interpretation of these results is that top-down frontoparietal connectivity is a marker of global loss of consciousness, including primary consciousness (e.g., Mashour, 2014 ). However, given that the reduction in top-down connectivity has also been observed under ketamine ( Lee et al., 2013 ), during which patients often report vivid dream-like experiences, it is plausible that the reduction of frontoparietal connectivity could instead indicate loss of self-awareness.
Cognitive neuroscience studies of lucid dreaming are uniquely placed to contribute to this question because the comparison of lucid REM sleep to non-lucid REM sleep is perhaps the only contrast that allows for a direct comparison between the global loss and recovery of reflective consciousness independently of global shifts in primary consciousness, arousal or vigilance state. Thus, the question of whether lucid REM sleep is associated with altered connectivity between frontal and parietal cortices has implications for several broad questions in the cognitive neuroscience of consciousness. Future studies evaluating changes in effective connectivity during lucid REM sleep dreaming, and in particular changes in top-down frontoparietal connectivity, would be valuable.
4. Brain lesions and lucid dreaming
In the neurological literature, to our knowledge only one paper has reported changes in lucid dreaming as a result of neurological insult or brain lesions ( Sagnier et al., 2015 ). The paper describes two case reports of young patients who reported lucid dreams following unilateral ischemic stroke to the left mediodorsal thalamus. The first patient was a 26-year-old female with a left anterior and mediodorsal thalamic stroke in an area supplied by the premamillary artery, as revealed by MRI scans. She reported frequent lucid dreams in the early morning hours, along with increased nightmares and nocturnal awakenings. She also reported that her lucid dreams mostly involved the hospital and medical staff that she encountered during her hospitalization, and included catastrophic events such as helicopter crashes and hyper-aggressive behaviors. The second patient was a 36-year-old male with a left mediodorsal thalamic stroke in the area supplied by the paramedian artery as revealed by MRI scans. He reported frequent lucid dreams following the stroke, which also tended to occur in the early morning hours, along with increased nocturnal awakenings, but without an increase in nightmares. Lucid dreams subsided after one month for both patients.
Lucid dreams have not previously been reported following either unilateral or bilateral thalamic stroke. However, loss of neurons in the anterior and dorsomedian thalamic nuclei that occurs in familial fatal insomnia is associated with loss of nocturnal sleep as well as oneiric ‘intrusions’ during wakefulness ( Montagna, 2005 ; Raggi, Cosentino, Lanuzza and Ferri, 2010 ). Furthermore, hypersomnia and irregular sleep are frequently reported following paramedian thalamic stroke ( Hermann et al., 2008 ; Luigetti et al., 2011 ). These clinical features could be manifestations of disruption of the intralaminar and midline thalamic nuclei located in the mediodorsal thalamus, which are part of the brain’s arousal network ( Van der Werf, Witter and Groenewegen, 2002 ). Thus, one possibility is that lucid dreams in these patients could have partly resulted from increased or abnormal functioning of the brain’s arousal systems during sleep, which is consistent with the reported increase in nocturnal awakenings. Both patients also reported that their dreams contained highly anxious and emotional content, which could reflect abnormal connectivity between these thalamic nuclei and limbic structures with which they are densely connected ( Van der Werf et al., 2002 ). The highly emotional or disturbing content may have also contributed to lucid dreaming, as these types of experiences could induce individuals to question whether the explanation for such surprising or frightening experiences is that they are dreaming ( LaBerge and Rheingold, 1990 ).
A systematic study of the incidence of lucid dreams following thalamic strokes is lacking. Particularly given that the lucid dreams subsided after a relatively short duration (one month) for both patients, it is possible that lucid dreaming as a consequence of thalamic strokes has been under-reported and/or overlooked. More generally, a large-scale study of the occurrence of lucid dreaming in neuropsychological cases has not been undertaken and would be a welcome addition to the literature. The converse case, of a brain lesion causing loss of lucid dreaming in an individual who regularly experiences lucid dreams has also to our knowledge not been reported. Such a case would likely be very unusual, particularly considering the small percentage of individuals who experience lucid dreams spontaneously with high frequency. However, if such a case were identified it could be informative to the neurobiology of lucid dreaming.
5. Pharmacological induction of lucid dreaming
A main target of research is to develop methods to make the lucid dream state more accessible. Indeed, reliable techniques to induce lucid dreams are needed for it to be effectively used in both clinical and scientific applications. Evidence suggests that lucid dreaming is a learnable skill ( LaBerge, 1980a ) that can be developed by training with various induction strategies ( LaBerge and Rheingold, 1990 ; Price and Cohen, 1988 ; Stumbrys, Erlacher, Schadlich and Schredl, 2012 ). These include training in prospective memory techniques ( LaBerge and Rheingold, 1990 ), which can be further aided by application of external sensory cues ( LaBerge and Levitan, 1995 ; LaBerge, Levitan, Rich and Dement, 1988 ; LaBerge, Owens, Nagel and Dement, 1981b ) and/or interrupting sleep with short periods of wakefulness ( Aspy, Delfabbro, Proeve and Mohr, 2017 ; LaBerge, 1980a ; LaBerge, Phillips and Levitan, 1994 ; Stumbrys et al., 2012 ). Within cognitive neuroscience, studies have evaluated pharmacological as well as non-invasive brain stimulation approaches to lucid dream induction. In this section, we review studies that have taken a pharmacological approach to lucid dream induction and in the next section we review electrical brain stimulation studies.
5.1. Effects of Acetylcholinesterase inhibitors (AChEIs) on lucid dreaming
As discussed above, lucid dreaming is associated with increased cortical activation ( LaBerge et al., 1981a ), which reaches its peak during phasic REM sleep. Given the relationship between phasic REM sleep and lucid dreaming, as well as the role of Acetylcholine (ACh) in REM sleep regulation (e.g., Amatruda, Black, McKenna, McCarley and Hobson, 1975 ; Velazquez-Moctezuma, Shalauta, Gillin and Shiromani, 1991 ), agents acting on the cholinergic system have received particular interest. In an initial pilot study, LaBerge (2001) evaluated the effect of donepezil (Aricept), an Acetylcholinesterase inhibitor (AChEI), on lucid dreaming in a small group of participants (N=10) who reported prior experience with lucid dreaming. On each night, participants received either 0 mg (placebo), 5 mg, or 10 mg of donepezil, with the dose order counterbalanced across the three nights of the experiment. Nine of the ten participants (90%) reported at least one lucid dream on donepezil, while only one participant reported a lucid dream on the placebo dose.
Following on these results and additional pilot research, LaBerge, LaMarca and Baird (2018b) conducted a double blind, placebo-controlled study in a large group of participants (N=121) with high dream recall and an interest in lucid dreaming. The first goal of the study was to quantify the size and reliability of the effect of AChEI on lucid dreaming. The second goal was to test the effectiveness of an integrated lucid dream induction protocol that combined cholinergic stimulation with other methods, including sleep interruption and the Mnemonic Induction of Lucid Dreams (MILD) technique, which trains participants to use prospective memory to induce lucid dreams ( LaBerge, 1980a ; LaBerge and Rheingold, 1990 ). Participants were randomly assigned counterbalanced orders of three doses of galantamine (0 mg=placebo, 4 mg, and 8 mg), an AChEI that is readily accessible, fast acting and has a mild side effect profile. On three consecutive nights, participants awoke approximately 4.5 hours after lights out (after approximately the 3 rd REM cycle), recalled a dream, ingested the capsules and stayed awake for at least 30 minutes. Participants then returned to sleep practicing the MILD technique. After each subsequent awakening, participants rated their dreams on a range of variables including lucidity, recall, vividness, bizarreness, complexity, affect, cognitive clarity, metacognition and control. Full reports of lucid dreams were also collected.
Galantamine was found to significantly increase the frequency of lucid dreaming in a dose-related manner. Increased incidence of lucid dreaming was observed for both 4 mg (27% of participants) and 8 mg (42% of participants) doses compared to 14% of participants for the active placebo procedure (which included sleep interruption and MILD). Galantamine was also found to be associated with significantly increased sensory vividness and environmental complexity, which might be expected given the general intensification of REM sleep, and associated dreaming, triggered by cholinergic stimulation ( Riemann, Gann, Dressing, Muller and Aldenhoff, 1994 ).
Another recent double blind, placebo-controlled study conducted by Sparrow, Hurd, Carlson and Molina, 2018 ) also tested the effect of galantamine on lucid dreaming. 35 participants completed an eight-night study that tested the effect of 8 mg of galantamine paired with 40 minutes of sleep interruption (termed “Wake-Back-to-Bed” (WBTB) in the study). The study additionally tested combining galantamine with middle-of-the-night meditation and the imaginary reliving of a distressing dream (termed meditation and dream reliving or MDR; Sparrow, Thurston and Carlson, 2013 ). The study included pre- and post-baseline nights and six conditions: 1) WBTB; 2) WBTB + placebo; 3) WBTB + galantamine; 4) MDR; 5) MDR + placebo; and 6) MDR + galantamine. MDR conditions matched the 40 minutes of sleep interruption in the WBTB conditions. Lucid dreams were measured with self-reports on the dream lucidity scale (DLS; Sparrow et al., 2013 ), which in this study included three categories: 0=non-lucid, l=pre-lucid (which included either “questioning things in the dream without actually concluding that you were dreaming” or “doing things that are ordinarily impossible to do”), or 2=lucid.
Both galantamine conditions (WBTB + galantamine; MDR + galantamine) significantly increased self-ratings of lucidity on the DLS compared to the other conditions. However, no significant difference was observed between WBTB + galantamine and MDR + galantamine. The number of participants who had a lucid dream in each condition was not reported, only the conditional means for the DLS, which reflects the effect collapsed across both pre-lucid and lucid dreams. We therefore contacted the authors for this information in order to compare the results of this study to the study by LaBerge et al. (2018b) . 9% of participants reported a lucid dream in the WBTB + placebo condition and 11% in the MDR + placebo, whereas 40% of participants reported a lucid dream in the WBTB + galantamine and 34% in the MDR + galanatamine (S. Sparrow, personal communication, December 17, 2018). Overall, therefore, these results are comparable to the effect of 8 mg galantamine observed by LaBerge and colleagues.
5.2. Effects of L-alpha glycerylphosphorylcholine (α-GPC) lucid dreaming
In contrast to the positive findings for AChEIs, a double blind randomized placebo-controlled study found no significant effect of 1200 mg of the ACh precursor L-alpha glycerylphosphorylcholine (α-GPC) on the frequency of lucid dreams in 33 participants with varying degrees of lucid dreaming experience ( Kern, Appel, Schredl and Pipa, 2017 ). One interpretation of this result is that, in contrast to AChEIs, α-GPC is not effective for inducing lucid dreams, perhaps due to differences in the neurobiological effects of choline—an ACh precursor—and AChEIs. However, participants in this study appear to have received no training in mental set, such as recalling and attending to dreams in an effort to become lucid. Thus, an alternative, not mutually exclusive, interpretation is that training in at least the minimal mental set for lucid dream induction is needed for pharmacological (and other) interventions to effectively increase the frequency of lucid dreams.
5.3. General discussion of pharmacological induction of lucid dreaming
Overall, these data provide strong initial evidence that cholinergic enhancement with AChEls, and galantamine in particular, facilitates a state of the brain favorable to lucid dreams. However, a limitation of all pharmacological studies on lucid dreaming performed to date is that they were not conducted in a sleep laboratory and there was therefore no validation of lucid dreams with eye-signaling methods. It will be important to follow up these results with sleep laboratory studies to objectively validate lucid dreams with polysomnographic recording. Such studies would also be valuable to investigate the physiological effects of galantamine on the brain during REM sleep, and which effects are predictive of lucidity. Given the high success rate of lucid dreams in the combined induction protocol with cholinergic stimulation reported by LaBerge, LaMarca and Baird (2018) and Sparrow et al. (2018) , studies of galantamine have the potential to enable efficient collection of large sample sizes in electrophysiological and neuroimaging studies of lucid dreaming.
The mechanism by which AChEls facilitate lucid dreams remains unclear. There are several, not mutually exclusive, possibilities, including increasing REM sleep intensity/phasic activation, influencing the brain regions/networks associated with lucid dreaming, and influencing cognitive processes associated with becoming lucid. In general, it is known that AChEls inhibit the metabolic inactivation of ACh by inhibiting the enzyme acetylcholinesterase (AChE), leading to accumulation of ACh at synapses. Furthermore, ACh and its agonists as well as AChE and its antagonists are involved in the generation of REM sleep ( Amatruda et al., 1975 ; Gillin et al., 1985 ; Velazquez-Moctezuma et al., 1991 ). For example, evidence suggests that REM sleep is controlled by cholinergic neurons in the brainstem ( Baghdoyan, 1997 ; Hernandez-Peon, Chavez-Ibarra, Morgane and Timo-Iaria, 1963 ), and studies have observed that microinjection of the ACh agonist carbachol in the pontine area of the brainstem results in REM sleep in both humans and animals ( Amatruda et al., 1975 ; Baghdoyan, 1997 ). Administration of galantamine has been associated with increased phasic activity and shortened REM latency ( Riemann et al., 1994 ). The increased frequency of lucid dreams associated with AChEls could therefore plausibly be related to its effects on cholinergic receptors during REM sleep, leading to longer, intensified REM periods with increased phasic activity, which, as noted above, has been found to be associated with lucid dreams ( LaBerge et al., 1981a ).
Beyond intensification of REM sleep/phasic activation, AChEls might also directly act on cognitive processes associated with lucidity and their neural underpinnings. One key question is whether AChEIs could facilitate lucid dreaming through increasing activation within the network of frontopolar-temporo-parietal areas observed in the neuroimaging studies of Dresler et al. (2012) and Baird et al. (2018a) . The relationship between cholinergic modulation and frontoparietal activation is complex and depends on the task context and population under study (see Bentley, Driver and Dolan, 2011 for a review). However, pro-cholinergic drugs in general tend to increase frontoparietal activity in conditions in which these areas show low baseline activation, which is thought to reflect increased attentional-executive functions ( Bentley et al., 2011 ). Given that frontoparietal activity is typically suppressed during REM sleep ( Braun et al., 1997 ; Maquet et al., 1996 ), it is plausible that AChEIs could increase activation within this network during REM sleep dreaming.
Notably, AChEls are also prescribed to manage the cognitive symptoms of Alzheimer’s disease ( Koontz and Baskys, 2005 ). Theoretically, another way that AChEls could facilitate lucid dreams is therefore through their effect on memory. For example, AChEIs could enhance the ability to remember to recognize that one is dreaming (a form of prospective memory), which is the core of the MILD technique for lucid dream induction that participants engaged in during the study by LaBerge, LaMarca and Baird (2018b) . However, evidence for cognition enhancing effects of AChEls in healthy subjects, particularly for single doses, is sparse ( Dresler et al., 2018 ), rendering the theoretical possibility of a memory-mediated effect on dream lucidity unlikely. Overall, it remains unclear whether galantamine also exerts a direct influence on cognitive processes associated with lucidity, and the MILD technique in particular, or whether it merely optimizes the physiological conditions for such techniques.
One last possibility is that AChEIs could influence lucidity indirectly by affecting other neuromodulators. For example, evidence suggests that AChEls also increase systemic norepinephrine and dopamine ( Cuadra, Summers and Giacobini, 1994 ; Giacobini, Zhu, Williams and Sherman, 1996 ). It is therefore possible that the increase in lucid dreams associated with AChEI might instead be directly linked to aminergic modulation that occurs as a result of the increases in ACh. Additional research will be needed to understand how the neuromodulatory changes caused by AChEIs stimulate lucid REM sleep dreaming. Studies using Positron Tomography (PET) or pharmaco-fMRI would be valuable to address this issue.
Several other pharmacological substances have been suggested to increase the frequency of lucid dreams, including various types of supplements and drugs (e.g., Yuschak, 2006 ). For instance, recreational drugs such as alcohol, cocaine and cannabis have been reported to be associated with lucid dreaming. These substances suppress REM sleep ( Roehrs and Roth, 2001 ; Schierenbeck, Riemann, Berger and Hornyak, 2008 ), which leads to a phenomenon referred to as REM rebound, in which longer REM sleep periods occur following REM sleep suppression ( Vogel, 1975 ). Intensified, prolonged REM sleep as a result of REM rebound could potentially increase lucid dream frequency, particularly in individuals predisposed to have lucid dreams or with prior experience in lucid dreaming. Another example is LSD, since there is some evidence that it can prolong REM sleep periods at some doses ( Muzio, Roffwarg and Kaufman, 1966 ), which could potentially be favorable to lucid dreaming. To our knowledge, however, no studies have systematically evaluated whether these substances, or other substances not reviewed here, increase the incidence of lucid dreams. Furthermore, it is prudent to remain cautious about such claims given the placebo effect. Placebo-controlled studies will be essential to substantiate any purported effects of pharmacological substances on lucid dreaming.
6. Induction of lucid dreams with transcranial electrical brain stimulation
Two studies have attempted to induce lucid dreams through transcranial electrical stimulation of the frontal cortex during REM sleep. Stumbrys, Erlacher and Schredl (2013b) tested direct current stimulation and Voss et al. (2014) tested alternating current stimulation. We review each of these studies in turn.
6.1. Effects of frontal transcranial direct current stimulation on lucid dreaming
In an investigation of the effect of transcranial direct current stimulation (tDCS) on lucid dreaming, Stumbrys et al. (2013b) applied either tDCS or sham stimulation (counterbalanced across nights) over a frontolateral scalp region in 19 participants. Stimulation was delivered through two pairs of electrodes with anodes at positions F3 and F4, respectively, and cathodes at the supraclavicular area of the same side. Stimulation was applied during all REM sleep periods except the first for two consecutive nights in the sleep laboratory. In total, 109 stimulations were performed, after which participants were awakened to complete a dream report.
Compared to sham stimulation, tDCS resulted in a small numerical increase in self-ratings of the unreality of dream objects as assessed by the Dream Lucidity Questionnaire (DLQ; Stumbrys et al., 2013b ). Post-hoc analyses revealed that this effect was seen only in subjects with a high baseline frequency of lucid dreams, but not in participants with little or no lucid dreaming experience. However, tDCS did not significantly increase the number of dreams rated by judges to have a clear indication of lucidity: seven dreams in total, three from sham stimulation and four from tDCS stimulation. Furthermore, only one lucid dream in total was confirmed by eye signaling, which was in the stimulation condition. Given a difference of only one lucid dream between stimulation and sham conditions for either of these two assessment criteria, overall frontal stimulation with tDCS as tested in this study does not appear to be a reliable method for inducing lucid dreams.
6.2. Effects of frontal transcranial alternating current stimulation on lucid dreaming
A study by Voss et al. (2014) reported a more pronounced increase in lucid dreaming by applying transcranial alternating current stimulation (tACS) in the gamma frequency band over the frontal cortex. The study tested 27 participants for up to four nights in the sleep laboratory. tACS was applied for 30 seconds after two minutes of uninterrupted REM sleep to frontolateral scalp regions at several different frequencies (2, 6, 12, 25, 40, 70 or 100 Hz or sham stimulation). Stimulation was delivered through two pairs electrodes with anodes at positions F3 and F4, respectively, and cathodes over the mastoids close to TP9 and TP10, respectively. Participants were then awakened and completed a dream report and the Lucidity and Consciousness in Dreams (LuCiD) scale ( Voss et al., 2013 ). The LuCiD scale measures dream content on several different factor-analytically derived dimensions, including insight, control, thought, realism, memory, dissociation and affect.
The authors reported they were able to induce lucid dreams with a success rate of 58% with 25 Hz stimulation and 77% with 40 Hz stimulation. However, it is important to note how lucid dreams were classified in this study: instead of assessment of lucid dreams with eye signaling, self-report, or through statistical analysis of judges’ ratings of dream reports, as in Stumbrys et al. (2013b) , dreams were assumed to be lucid if subjects reported “elevated ratings (>mean + 2 s.e.) on either or both of the LuCiD scale factors insight and dissociation”. While dissociation scores (i.e., “seeing oneself from the outside” or a “3 rd person perspective”) have been found to be increased in lucid dreams before ( Voss et al., 2013 ), dissociation in the sense of adopting a 3 rd person perspective has never been considered a defining feature of lucid dreams per se (e.g., DeGracia and LaBerge, 2000 ; Gackenbach and LaBerge, 1988 ; Green, 1968 ; LaBerge, 1985 , 1990 ). It is therefore controversial to classify dreams as lucid based solely on higher ratings of dissociation.
The insight subscale corresponds more closely to the standard definition of lucid dreams adopted by other researchers as well as the general public. Mean ratings in the insight subscale increased from approximately 0.1-0.2 in the sham stimulation to 0.5-0.6 in the 25 Hz or 40 Hz stimulation conditions, similar to the effects in the dissociation subscale, which was approximately 0.9-1.2. However, the scale anchors ranged from 0 (strongly disagree) to 5 (strongly agree), indicating that, on average, in both the 25 Hz and 40 Hz stimulation conditions, participants disagreed that their dreams had increased insight. Moreover, in the original validation of the LuCiD scale, non-lucid dreams scored on average at 0.3 or 0.8 on the insight scale (depending on whether the assessment was laboratory or survey based), whereas lucid dreams scored at 3.2 or 3.5 ( Voss et al., 2013 ). Thus, though significantly stronger in relation to sham simulation, mean responses of 0.5-0.6 in the 25 and 40 Hz stimulation conditions were much lower than the values reported for lucid dreams in the validation study, and still on the non-lucid (disagree that the dream contains insight) end of the scale spectrum in absolute values. Overall, therefore, the results of this study appear to be comparable to the tDCS finding of Stumbrys et al. (2013b) in that prefrontal tACS gamma band stimulation induced numerical increases in some measures of dream content, but does not appear to have lead to increases in the number of lucid dreams when defined in the traditional sense of being aware of dreaming while dreaming.
6.3. General discussion of induction of lucid dreaming with electrical brain stimulation
In summary, studies examining induction of lucid dreams with electrical brain stimulation (tDCS and tACS) have thus far observed intriguing effects on dream cognition, but a method for reliably inducing lucid dreams by electrical stimulation of the brain is still yet to be identified. We think this is a particularly interesting direction for upcoming work. Future studies might consider stimulating a wider number of brain areas and different types of stimulation. For example, transcranial magnetic stimulation (TMS) is another method of noninvasive brain stimulation that could be used to attempt to induce lucid dreams that has not yet been examined ( Noreika, Windt, Lenggenhager and Karim, 2010 ; Mota-Rolim et al., 2010 ). In contrast to tACS or tDCS, high-frequency repetitive TMS (rTMS) stimulation sequences can be used to increase neuronal excitability in focal areas of the cortex (e.g., Hallett, 2007 ). Several practical challenges with application of TMS during sleep include the auditory artifacts produced by the TMS coil as well as tactile sensations on the scalp during stimulation, which may lead to awakenings. However, noise masking has been used to prevent subjects from hearing the clicks associated with TMS pulses (e.g., Nieminen et al., 2016 ), and scalp sensations could be decreased, for example, by reducing stimulation intensity or potentially by application of a topical anesthetic cream.
Studies of electrical brain stimulation have also thus far only tested a small part of the parameter space and there are many other stimulation protocols that appear to be worth evaluating. For example, given the neuroimaging results reviewed above, synchronous frontoparietal tACS—in which synchrony is increased between frontal and parietal regions by simultaneous stimulation of these regions (e.g., Violante et al., 2017 )—is another stimulation method that would be interesting to examine. Finally, as noted above, these methods are likely to maximize their effect when participants are trained in at least the minimal mental set for lucid dream induction, which was not done in either the study by Voss et al. (2014) or Stumbrys et al. (2013b) .
7. Lucid dreaming as a methodology for the cognitive neuroscience of consciousness
Psychophysiological studies of non-lucid dreams have been used to study neural correlates of conscious experiences during sleep ( Perogamvros et al., 2017 ; Siclari et al., 2017 ). However, there are significant limitations to this approach. Specifically, while methods such as “serial awakenings” are useful for contrasting the global presence vs. absence of dream experience during sleep ( Siclari et al., 2017 ), it is an inefficient method to collect data on specific conscious contents during sleep. For instance, given that non-lucid dreamers usually have no control over their dream content, these studies typically employ a shot-in-the-dark approach, in which large numbers of sleep recordings are made and small subsets of data in which the content of interest appears by chance are extracted. Additionally, and perhaps most importantly, establishing temporally precise correlations between retrospective dream reports and physiological measurements is a substantial challenge. Lucid dreaming provides ways to overcome several of these challenges. For example, as mentioned above, lucid dreamers can conduct specific tasks within REM sleep dreams, enabling experimental control over dream content. Furthermore, as noted, participants can be trained to time-stamp the onset and offset of particular content or actions with eye movement signals, which provides a way to obtain more precise correlations between conscious experiences and physiological measurements during sleep ( Dresler et al., 2011 ; LaBerge, 1990 ; LaBerge, Greenleaf and Kedzierski, 1983 ). In this section we briefly summarize several recent studies that illustrate how lucid dreaming can be used as a methodology in the cognitive neuroscience of consciousness.
7.1. Activation of sensorimotor cortex during dreamed movement in REM sleep
Dresler et al. (2011) used lucid dreaming to test whether a motor task performed during dreaming elicits neuronal activation in the sensorimotor cortex. Participants made a series of dreamed left and right hand clenches in their lucid dreams and marked the start and end of the sequences of clenches with LRLR eye movements. Specifically, participants clenched their left hand in the dream ten times, then signaled LRLR, then clenched their right hand in the dream ten times, then signaled LRLR, and continued repeating this sequence for as long as possible until awakening. Additionally, participants performed an executed hand-clenching task as well as imagined hand-clenching task during wakefulness for comparison. Six participants with prior experience in lucid dreaming participated in the study. Of these, two participants succeeded in performing the task during lucid REM sleep: one under simultaneous EEG-fMRI (in two different signal-verified lucid dreams) and one under combined EEG-near-infrared spectroscopy (NIRS) recording (again in two different signal-verified lucid dreams).
Contrasting left vs. right fist clenches, increased BOLD signal in the contralateral sensorimotor cortex was observed in both lucid dreams of the fMRI experiment, as well as in waking and imagination conditions. Compared to executed hand clenches during wakefulness, however, BOLD signal increases during dreaming in contralateral sensorimotor cortex were more localized, which could indicate either weaker activation or more focal activation of hand areas only. BOLD signal fluctuations during dreaming were approximately 50% of those observed for executed hand clenches during wakefulness. Correspondingly, the NIRS data showed increased oxygenated hemoglobin and decreased deoxygenated hemoglobin in the contralateral sensorimotor cortex during dreamed hand clenching. This hemodynamic response was also observed in the supplementary motor area, which is involved in the timing, monitoring and preparation of movements ( Goldberg, 1985 ). This differed with the fMRI data in which no significant differences in the supplementary motor area were found. Interestingly, during dreamed hand clenching, hemodynamic responses were smaller in the sensorimotor cortex but of similar amplitude in the supplementary motor area when compared to overt motor performance during wakefulness.
Overall, these data suggest that the pattern of brain activation observed during dreamed motor execution overlaps with motor execution during wakefulness. However, given the different patterns of activation, the data may also suggest that the interactions between the supplementary motor area, somatosensory and sensorimotor cortex differs between REM sleep dreaming and waking. The authors suggest that the reduced activation in sensorimotor cortex could be due in part to the lack of sensory feedback as a result of REM sleep atonia. However, given that this study consisted of two case reports—one for each imaging modality—the data should be interpreted cautiously. Clarification of the neural correlates of dreamed motor activity, as well as clarification of any differences in this network compared to overt motor performance, awaits larger-scale group-level studies. Nevertheless, this experiment provides a proof-of-concept that neuroimaging of specific dream content can be accomplished using lucid dreaming as a methodology.
7.2. Voluntary control of central apneas during REM sleep dreaming
A similar type of experiment was recently undertaken by Oudiette et al. (2018) to examine respiratory behavior during REM sleep dreaming. Specifically, the researchers used lucid dreaming to investigate whether irregular breathing during REM sleep has a cortical origin and whether such breathing patterns can reflect the mental content of dreams. This research follows up on a study from LaBerge and Dement (1982) which observed tachypnea during volitional rapid breathing and apnea during voluntary breath holding during lucid REM sleep dreaming. Polysomnography and respiration were recorded during early morning naps from 21 patients with narcolepsy who reported frequent lucid dreams. Participants were instructed to modify their dream scenario so that it involved vocalizations or an apnea (e.g., diving under water)—two respiratory behaviors that purportedly require a cortical control of respiration ( McKay, Adams, Frackowiak and Corfield, 2008 ). Participants signaled the onset of lucidity as well as the start and end of the respiration tasks with LRLR eye movement signals. Participants also performed the task during waking by actually executing the respiratory task as well as during waking imagination. In total, 32 lucid REM sleep naps were included in the analysis. Physiological data was scored for the presence of central apneas, which were defined as “cessation of nasal flow for more than 10 s in the absence of cyclic thoracic and abdominal movements” as well as preparatory breaths preceding the central apnea.
In 16 out of the 32 signal-verified lucid dreams, the physiological data showed the expected respiratory behavior (e.g., a central apnea, in some cases including preparatory breaths), which was appropriately marked by LRLR signals and confirmed by dream reports of becoming lucid and executing the task. In the remainder of cases, participants either failed to control the dream (N=2) or appropriately execute the LRLR signals (N=2), or there was incongruence between the report and the data (participants either did not recall performing the task (N=8) or there was no physiological evidence of the task despite a report of completing the task (N=4)). As the authors discuss, the incongruences between reports and physiological data may in part have been attributable to the fact that reports were obtained at the end of the 30-minute nap sessions rather than awakening subjects directly after completing the task. Thus, in some cases this resulted in a long delay between the dream task and the report. Despite the presence of these ambiguous cases, overall the data show that voluntarily control of central apneas during REM sleep occurred in a majority of participants.
As the authors discuss, the pons is hypothesized to regulate cessation of breathing, which assimilates input from supra-brainstem structures and inhibits medullary respiratory neurons ( McKay et al., 2008 ). Thus, they conclude that the fact that voluntary control of central apneas is possible during REM sleep suggests that this cortico-pontine drive is preserved during REM sleep. However, it remains unclear from this study to what extent non-lucid dreams containing this type of mental content (for example, diving under water), would result in central apneas. Indeed, as the authors note, some participants reported that they voluntarily held their breath when diving under water in the dream even though they did not feel that they had to. In general, it remains unclear whether central apneas as observed in the study are linked to the voluntary control of respiration enabled during lucid dreams in particular or whether they systematically occur in dreams with this type of content/scenario. In either case, however, the data support the conclusion that voluntarily control of central apneas is possible during REM sleep.
7.3. Smooth pursuit eye movements during visual tracking in REM sleep dreaming
One last recent example of the use of lucid dreaming as methodology in cognitive neuroscience is provided by LaBerge, Baird and Zimbardo (2018a) , who addressed the question of whether smooth pursuit eye movements (SPEMs) occur during tracking of a slowly moving visual target during lucid REM sleep dreaming. Seven participants with high reported dream recall and frequency of lucid dreams participated in the study. Participants marked the moment of lucidity as well as the initiation and completion of the tracking tasks with LRLR eye movement signals. After making the LRLR signal, participants performed one of two eye-tracking tasks: circle tracking or one-dimensional movement tracking on the horizontal meridian. For each tracking task, participants followed the tip of their thumb with their gaze as they traced the pattern. Participants performed the tracking tasks in three conditions: 1) lucid REM sleep dreaming (“dreaming”), 2) awake with eyes open (“perception”) and 3) tracking the imagined movement while awake with eyes closed (“imagination”). Eye movements were recorded with direct current EOG and subjected to a validated algorithm that classifies saccades, fixations and smooth pursuit eye movements ( Komogortsev and Karpov, 2013 ).
The results revealed that intentional slow tracking of visual motion (of both circles and lines) during lucid REM sleep dreams results in SPEMs. Pursuit eye movements in REM sleep dreams did not significantly differ from pursuit during waking perception, and both were characterized by high pursuit ratios and low saccade rates. In contrast, tracking in imagination was characterized by low pursuit with frequent saccadic intrusions. A Bayesian classification model that included pursuit ratio and saccade rate discriminated both REM sleep dreaming and perception from imagination with greater than 98% accuracy.
Together, these findings help to address several broad questions within cognitive neuroscience and sleep research. First, the data provide empirical evidence for a difficult to test question that has been asked at least since Aristotle: “Are dreams more like perception or imagination?” ( Nir and Tononi, 2010 ). Based on the smooth tracking behavior, the findings of this study suggest that, at least in this respect, the visual quality of REM sleep dream imagery is more similar to waking perception than imagination. Second, the findings help to address a longstanding question in the psychophysiology of sleep since the discovery of REM in the 1950’s: whether the eye movements of REM sleep track the gaze of the dreamer—the so-called “scanning hypothesis” (for a review, see Arnulf, 2011 ). By demonstrating that individuals can trace circles and lines with their gaze while in EEG-verified REM sleep, which can be recorded with EOG, the data provide unique evidence that shifts in the perceived gaze direction in dreams give rise to the appropriate corresponding eye movements. This is consistent with the view that a subset of eye movements during REM sleep are linked to the direction of subjective gaze during dreams. Lastly, the results also provide a novel source of data for a central research question on the topic of smooth pursuit in humans and non-human primates that dates back at least forty years. Specifically, an enduring question has been whether a physical stimulus and/or retinal image motion is necessary to drive the neural circuitry of smooth pursuit ( Spering and Montagnini, 2011 ). By demonstrating that sustained SPEMs can be elicited in the absence of visual input to the cortex, as is the case during REM sleep, the findings provide strong evidence that neither a physical motion stimulus nor readout of retinal image motion are necessary for SPEMs.
7.4. General discussion of lucid dreaming as experimental methodology
Altogether, these studies illustrate the potential of lucid dreaming as an experimental methodology for the study of consciousness in general and REM sleep dreaming in particular. The fact that lucid dreamers can exercise volitional control over their actions while dreaming and conduct experiments from within EEG-verified REM sleep dreams opens up the ability to perform experiments that would otherwise be difficult or impossible to conduct. This methodology also has the potential to more efficiently target specific research questions. As discussed above, lucid dreaming enables both experimental control over the content of dreams as well as a way to establish precise psychophysiological correlations between the contents of consciousness during sleep and physiological measures in a way that is not possible in studies of non-lucid dreaming. In sum, lucid dreaming provides a methodology that allows for new ways of studying the relationship between consciousness and neurophysiological processes, and, as the above studies highlight, shows emerging potential for research on consciousness in sleep science.
8. Clinical cognitive neuroscience of lucid dreaming
Research on lucid dreaming is not only relevant to the neuroscience of consciousness but could also have clinical implications. While a detailed treatment of the clinical applications of lucid dreaming is beyond the scope of this review (see e.g., Garfield, Fellows, Halliday and Malamud, 1988 ), here we briefly note several interesting applications and future directions with particular relevance for cognitive neuroscience.
8.1. Lucid dreaming in the treatment of persistent nightmares
The most researched application of lucid dreaming is to treat recurring nightmares ( Abramovitch, 1995 ; Holzinger, Klösch and Saletu, 2015 ; Spoormaker and Van Den Bout, 2006 ; Tanner, 2004 ; Zadra and Pihl, 1997 ). Lucid dreaming was included by the American Academy of Sleep Medicine in their therapy suggestions for nightmare disorder ( Morgenthaler et al., 2018 ). Conceptually, the idea is that becoming lucid during a nightmare should allow the dreamer to realize the content of the nightmare is not real and thus has no reason to be afraid, and to be able to exert control over the dream and/or work with the psychological content of the nightmare ( LaBerge and Rheingold, 1990 ; Mota-Rolim and Araujo, 2013 ). Neurocognitive models of nightmare generation suggest a hyper-responsivity of the amygdala coupled with a failure of prefrontal regions to dampen this activation ( Levin and Nielsen, 2007 ). Studying the influence of lucidity on nightmare resolution could therefore provide an interesting opportunity to study top-down regulation of amygdala activation, perhaps through reactivation of prefrontal regions ( Dresler et al., 2012 ).
A case study ( Zadra and Pihl, 1997 ), and one small, controlled pilot-study ( Spoormaker and Van Den Bout, 2006 ) have found that lucid dreaming therapy was effective in reducing nightmare frequency. Also a study of 32 patients who suffer from frequent nightmares found a slight advantage of lucid dreaming as add-on to Gestalt therapy compared to the latter alone ( Holzinger et al., 2015 ). In contrast, a larger online study did not find any additional effect of lucid dreaming therapy as an add-on to other cognitive-behavioral techniques, such as imagery rehearsal therapy ( Lancee, Van Den Bout and Spoormaker, 2010 ), although low power and high dropout rates (>70%) limited the scope of the conclusions. Controlled experiments with larger sample sizes are needed to further evaluate the potential usefulness of lucid dreaming for the treatment of recurring nightmares and related disorders, as well as to examine the neural mechanisms of these interactions.
8.2. Lucid dreaming and narcolepsy
Consistent with a potential beneficial role of lucid dreaming in recurring nightmares, patients with narcolepsy report that becoming lucid provides psychological relief ( Rak et al., 2015 ). Generally, narcolepsy patients experience longer, more complex, and more vivid dreams and more frequent nightmares than healthy subjects ( Mazzetti et al., 2010 ). Moreover, patients with narcolepsy report higher reflective consciousness within dreams ( Fosse, 2000 ) and accordingly a considerably increased lucid dreaming frequency ( Dodet et al., 2014 ; Rak et al., 2015 ). The mechanisms for this increased lucid dreaming frequency are not entirely understood yet: nightmares often lead to the insight into the current dream state, however it is currently unclear if an increased nightmare frequency causes an increased lucid dreaming frequency in narcolepsy, or if the strongly increased frequency of REM sleep in narcolepsy affects nightmares and lucid dreaming independently. In particular sleep onset REM (SOREM) episodes appear to prompt lucid dreams ( Dodet et al., 2014 ), which is in line with the dream lucidity-enhancing effects of the early morning “Wake-Back-to-Bed” procedure in healthy subjects. Of note, patients with narcolepsy make a clear distinction between their experiences of lucid dreaming and sleep paralysis ( Dodet et al., 2014 ), and the increased lucid dreaming frequency in this patient group does not appear to be associated with medication use (Rak et al., 2014). It has been speculated whether, potentially as a result of a considerably increased lucid dreaming experience, narcoleptic patients might exhibit different neurophysiological correlates of lucid dreaming ( Dodet et al., 2014 ).
8.3. Lucid dreaming as a model of insight in psychiatric conditions
Another area in which research on lucid dreaming might be applied is in psychiatric disorders in which patients suffer from lack of insight into their state. Indeed, there is considerable overlap between preliminary findings of brain regions related to insight into the dream state and brain regions impaired in psychotic patients with disturbed insight into their pathological state ( Dresler et al., 2015 ; Mota, Resende, Mota-Rolim, Copelli and Ribeiro, 2016 ). The concept of insight is becoming an increasingly important area in schizophrenia research (e.g., Baier, 2010 ), for example, where between 50 and 80% of patients diagnosed with schizophrenia have poor insight into the presence of their disorder ( Lincoln, Lullmann and Rief, 2007 ). Evidence suggests that poor insight in turn leads to more relapses, re-hospitalizations and poorer therapy success ( Mintz, Dobson and Romney, 2003 ). With regard to dreaming, lack of insight into the current state characterizes almost all dream experiences, with the exception of lucid dreaming. Thus, lucid dreaming could potentially be used as a model for at least some cognitive dimensions of insight ( David, 1990 ). In this context it is interesting to note that historical approaches to psychosis used the term lucid to denote the awareness of the patient into his or her illness ( Berrios and Markova, 1998 ). At the current time, these ideas remain highly speculative, but this appears to us to be an area worthy of additional research.
8.4. Using lucid dreaming to establish brain activity markers of self-awareness
Another potential clinical application of research on lucid dreaming is in the development of neuroimaging-based diagnostic markers of awareness. Such measures have the potential to improve the diagnosis and monitoring of patients who are unresponsive due to traumatic injury, aphasia, motor impairment, or other physical limitations. Neurobiological studies of lucid dreaming could provide information relevant to development of these brain activity markers since, in addition to assessing a patient’s capacity for primary consciousness (i.e., if a patient can see, hear or experience pain), an important clinical goal is to assess whether patients, who may nevertheless be unresponsive via behavioral assessment, are aware of themselves and their state ( Laureys, Perrin and Bredart, 2007 ). This information is critical to making appropriate therapeutic choices and determining prognosis ( Laureys et al., 2007 ). Research indicates that the bedside behavioral assessment of such patients is challenging and has a high rate of misdiagnosis (over 40% according to some studies) ( Laureys, Owen and Schiff, 2004 ; Schnakers et al., 2009 ). Accordingly, identification of a reliable brain activity marker of a patients’ capacity for self-awareness—which could be informed by studying the changes in brain activity between lucid and non-lucid REM sleep dreaming—is an important goal. Research on lucid dreaming has the potential to contribute a valuable source of data to this question since, as noted above, the contrast between lucid and non-lucid REM sleep is perhaps the only one currently known in which a global loss and recovery of self-awareness can be contrasted within the same vigilance state in healthy individuals.
9. The measurement of lucid dreaming in cognitive neuroscience research
In this last section, we review methodological issues and strategies in the measurement of lucid dreaming in cognitive neuroscience research. We review the validation of lucid dreaming both physiologically and with questionnaires, and discuss best-practice procedures to investigate lucid dreaming in the sleep laboratory.
9.1. Eye signaling methodology
The eye signaling methodology is the gold standard in lucid dreaming research, as it allows for objective confirmation of lucid dreams through the execution of pre-agreed upon sequences of eye movements recorded with the EOG during EEG-verified REM sleep ( Figure 1 ). While there are some types of research studies (e.g., field studies) where eye signaling is not possible or not applicable, we recommend considering this method as the standard practice for laboratory-based studies of lucid dreaming. In general, most recent studies have adhered to this convention; however, there is some confusion about how to properly instruct participants in executing the eye movements, which has resulted in studies implementing several different variations of the method. For instance, while some experimenters instruct participants to move their eyes left and right (e.g., Dresler et al., 2012 ), in other cases participants have been instructed to “scan the horizon” from left to right ( Dodet et al., 2014 ).
Differences in eye signaling instructions could partly account for the varying degrees of effectiveness in objectively identifying the eye signals across studies. For example, Dodet et al. (2014) reported that while three control participants and twelve narcoleptic patients reported making the eye signal in overnight EEG recordings, none of these could be objectively identified, and only about half of reported signals could be unambiguously identified from nap recordings. While it is possible that some of these instances represent cases where participants misremembered or misreported a lucid dream, it is likely that this high rate of ambiguous eye movement signals is partly attributable to the specific instructions that were given. Similarly, while the exact instructions provided to participants was not described, Voss et al. (2009) stated that they were unable to obtain reliable eye signals using the standard signaling method and therefore attempted to develop a novel signaling method employing two sets of eye-signals separated by a pause. Again, suboptimal results could plausibly be due to the instructions provided and the way the eye signals were executed by participants in the study.
One reason for the confusion and diverse instructions given for the eye signaling methodology is that a standardized set of instructions for making the signals has never been published. Here we report a simple set of instructions adapted from LaBerge et al. (2018a) that has been reported to yield nearly 100% correspondence between subjective reports of eye signals and objective EOG recordings. The instruction is as follows:
When making an eye movement signal, we would like you to look all the way to the left then all the way to the right two times consecutively, as if you are looking at each of your ears. Specifically, we would like you to look at your left ear, then your right ear, then your left ear, then your right ear, and then finally back to center. Make the eye movements without moving your head, and make the full left-right-left-right-center motion as one rapid continuous movement without pausing.
Looking at the ears is one of the critical aspects of the instruction, as it encourages full-scale eye movements without corresponding head movements. These extreme and rapid consecutive eye movements make the signals easy to discern in the horizontal EOG time series. The signals are of higher amplitude than typical REMs, and have a distinctive shape ( Figure 1 ) which can be identified with template matching ( LaBerge et al., 2018a ). To optimize execution of the eye signals, participants are given the opportunity to practice the signals in the laboratory while awake and connected to the EOG, with an opportunity to view the signals and receive feedback from the experimenter. Additionally, participants practice making the eye signals in any lucid dreams they have at home in the weeks leading up to the sleep laboratory visit, in order to gain experience with executing the signals.
9.2. Questionnaire assessment of lucid dreaming
A related issue concerns how the phenomenology of lucid dreaming should optimally be assessed in cognitive neuroscience research. Several questionnaires have been developed to assess changes in conscious experience during dreaming. For instance, the Metacognitive, Affective, Cognitive Experience questionnaire (MACE; Kahan, LaBerge, Levitan and Zimbardo, 1997 ; Kahan and Sullivan, 2012 ) was designed to assesses the monitoring and regulation of both cognitive and affective experience during both wakefulness and sleep. In its latest form, it consists of ten items, including four self-monitoring questions, three questions on self-reflective consciousness and three questions that target self-regulatory behaviors. Another measure, the Dream Lucidity Questionnaire (DLQ; Stumbrys et al., 2013b ), is designed to assess different aspects of lucidity within dreams. It consists of ten items, scored on a 5-point scale: 0 – not at all, 1 – just a little, 2 – moderately, 3 – pretty much, 4 – very much. The DLQ evaluates different types of awareness (awareness of dreaming, awareness that the physical body is asleep, awareness that dream characters and objects are not real, awareness of different possibilities) and control (deliberately choosing an action, changing dream events, dream characters, dream scenes, breaking physical laws). In a similar manner, the Lucidity and Consciousness in Dreams scale (LuCiD, Voss et al., 2013 ) includes eight subscales, derived from factor analysis of a sample of lucid and non-lucid dream reports, which assess various dimensions of dreaming experience and cognition: 1) Insight, 2) Control, 3) Thought, 4) Realism, 5) Memory, 6) Dissociation, 7) Negative emotion, and 8) Positive emotion.
All three questionnaires provide measures for evaluating how some content dimensions might differ across lucid and non-lucid dreams. However, as mentioned, questionnaires such as these are not sufficient to objectively establish whether a participant had a lucid dream. It is worth noting that only the DLQ in its first question (“I was aware that I was dreaming”) directly queries whether the dreamer was lucid, and the MACE was never intended for this purpose. Most studies using the DLQ and LuCiD questionnaires have collected a dream report from participants in addition to the questionnaire responses. We would like to emphasize the importance of this point: Instead of relying on the inference of lucidity solely from questionnaire measures of dream content, accurate assessment of lucidity can be facilitated by, in addition to using the eye signaling method, collecting a full dream report from participants. In making the dream report, participants are typically asked to describe in as much detail as possible the narrative of the dream, including the sequence of events and any thoughts, feelings or sensations that they experienced. In dream reports for lucid dreams, the participant is also asked to include a specific description of how they became lucid in the dream (for example, by noticing an oddity of an event, action or person in the dream, which is also known as “dream sign”) and also to explicitly note any eye signals made at the appropriate instances in the dream narrative.
Following the collection of a full open-ended dream report, further confirmation of lucidity and eye signaling can be accomplished with simple follow-up questions, including, for example: 1) “Were you aware of the fact that you were dreaming while you were dreaming?” (YES/NO), If YES: 2) “How confident are you that you became lucid?” (0-4 scale), 3) “Did you have a wake-initiated lucid dream (WILD) or a dream initiated lucid dream (DILD)?” (DILD/WILD), 4) “Did you make the eye movement signal to indicate that you became lucid?” (YES/NO), 5) “Please briefly describe how you became lucid.” These example questions are not meant to be exhaustive, but they illustrate the types of questions that in our view are useful to obtaining an accurate and thorough assessment of lucid dreams following collection of a full dream report.
9.3. Measurement of individual differences in lucid dreaming
Another related question facing researchers is how to measure individual differences in lucid dream frequency, which has been done in inconsistent ways, and could be improved in future research. A method used in several studies consists of an 8-point scale that asks participants to self-rate the frequency with which they experience lucid dreams, ranging from “never” to “several times per week” ( Schredl and Erlacher, 2004 ; Mota-Rolim et al., 2013 ). While this method provides a straightforward coarse assessment of an individual’s estimated frequency of lucid dreams that has shown high test-retest reliability ( Stumbrys, Erlacher and Schredl, 2013a ), a limitation is that it does not measure lucid dream frequency greater than several times per week. The scale could be improved by including additional categories on the higher end of the measure, including, for example, “Every night” and “Multiple times per night.” While individuals who experience lucid dreams on a nightly basis represent a very small percentage of respondents (according to Baird et al. (2018a) approximately one in one thousand), optimally this kind of instrument would enable a researcher to distinguish respondents that have lucid dreams once or several times a week from those that have them every night or multiple times per night. Indeed, these “virtuoso” lucid dreamers represent perhaps one of the most interesting populations for cognitive neuroscience studies of individual differences in lucid dreaming ( Baird et al., 2018a ).
A related method is to query the number of lucid dreams reported in a given period of time (i.e., the last 6 months), which has the advantage of asking participants to report a specific number rather than a coarse estimate. In principle, this method has the potential to be more accurate and to capture increased variance. However, some participants, particularly those with high lucid dream rates, may not be able to recall all instances of their lucid dreams within the requested time interval, and may therefore resort to heuristics when answering the question, making it akin to simply asking participants to report frequency using a multiple-choice scale. Furthermore, this method may not accurately assess lucid dream frequency over longer periods of time – i.e., an individual may normally experience lucid dreams frequently, but has not experienced them as frequently during the last queried interval of time. If using this approach, it is therefore advisable to also collect additional estimates of frequency, including the most lucid dream episodes experienced in any 6-month period (e.g., LaBerge et al., 2018b ).
A limitation of the above methods is that they do not measure variation in the length or degree of lucid dreams. Indeed, lucid dreams can range from a mere fleeting thought about the fact that one is dreaming followed by an immediate loss of lucidity or awakening, to extended lucid dreams in which an individual is able to engage in sequences of actions (e.g., LaBerge and Rheingold, 1990 ). Distinguishing between these different “levels” or subtypes of lucid dreams will likely be valuable to understanding observed differences (or lack of differences) in brain structural or functional measures associated with lucid dream frequency. Along these lines, several recent questionnaires have taken first steps to measure individual differences in specific characteristics of lucid dreaming. For example, the Lucid Dreaming Skills Questionnaire (LUSK) measures participants’ frequency of several different but inter-related aspects of awareness and control in lucid dreams ( Schredl, Rieger and Göritz, 2018 ). Another questionnaire, the Frequency and Intensity Lucid Dreaming Questionnaire (FILD) queries participants regarding the duration of their lucid dreams and various aspects of dream control, as well as the frequency with which they deliberately attempt to induce lucid dreams ( Aviram and Soffer-Dudek, 2018 ). In addition to providing data for individual differences studies, such questionnaires could also potentially be useful in selecting participants for sleep laboratory experiments of lucid dreaming, for example by selecting participants who report high levels of dream control in addition to frequent lucid dreams (in order to select participants who are more likely to be able to effectively make the eye signals or engage in experimental tasks during lucid dreams).
For all the above methods, important steps need to be taken to minimize measurement error, particularly to ensure that participants have a clear understanding of the meaning of lucid dreaming ( Snyder and Gackenbach, 1988 ). These include providing participants with a written definition of lucid dreaming, asking participants to provide written examples of their own lucid dreams to ensure clear understanding, as well as additional vetting through participant interviews ( Baird et al., 2018a ). Ultimately, however, basing the measurement of individual differences in lucid dreaming solely on self-report is not optimal. One way to further validate participant questionnaire responses would be to attempt to physiologically validate at least one lucid dream in the sleep laboratory for each participant. While additional validations such as this could potentially be valuable to incorporate in future studies, it is important to note that the estimated frequency of lucid dreaming would still depend on questionnaire assessment. Thus, approaches such as this do not obviate the reliance on questionnaire assessment.
An intriguing, though ambitious, method for deriving a measure of lucid dream frequency not dependent on questionnaire assessment would be to utilize home-based EEG recording systems to collect longitudinal sleep polysomnography data, from which estimates of lucid dreaming frequency could be derived from the frequency of signal-verified lucid dreams collected over many nights. However, this approach would only measure the frequency of signal-verified lucid dreams, and instances in which participants achieved lucidity but did not make the eye signal due to factors such as awakening, forgetting the intention, or lack of dream control would be missed by this procedure. There are many more points that could be addressed on the topic of questionnaire assessment of lucid dreaming frequency, but an extended analysis of this issue is beyond the scope of the present review.
9.6. General discussion of the measurement of lucid dreaming in cognitive neuroscience
In summary, cognitive neuroscience studies of lucid dreaming have at their disposal a unique set of rigorous methodological tools, including in particular the eye movement signaling method, which allows for the objective validation of lucid dreaming as well as precise time-stamping of physiological data. However, refinement of the instructions given to participants, as described above, could help further increase the reliability of the technique. Accurate phenomenological assessment of lucidity has been more mixed. In sleep laboratory studies using the eye signaling method, phenomenological reports are important but less critical due to the presence of an objective marker of lucidity. In contrast, in studies without eye signaling, accurate phenomenological assessment of whether an individual was lucid becomes essential, and inadequate or ambiguous measurement of lucidity can undermine the interpretability of the results. Questionnaire measures such as the DLQ ( Stumbrys et al., 2013b ) or LuCiD scale ( Voss et al., 2013 ) by themselves do not provide an unambiguous assessment of whether an individual had a lucid dream. Cognitive neuroscience research on lucid dreaming would greatly benefit from the further development of improved questionnaire measures for the validation of lucidity and the cognitive and experiential changes that accompany it, as well as further development of standardized measures for quantifying both the frequency and degree of lucidity. Overall, this brief discussion highlights the need for a set of standard operating procedures for both the phenomenological and objective sleep laboratory assessment of lucid dreaming.
10. Conclusion
Despite having been physiologically validated for approximately four decades, the neurobiology of lucid dreaming is still incompletely characterized. Most studies conducted to date have relied on small sample sizes, which limits the generalizability of the findings. Not surprisingly, the results of such underpowered studies are not consistent: almost every EEG study reports changes in spectral power in a different frequency band or brain area. Neuroimaging data on lucid dreaming is even sparser. Currently, there is only one fMRI study contrasting lucid and non-lucid REM sleep and it is a case study. Nevertheless, the results of this study converge with MRI studies that have evaluated individual differences in lucid dreaming frequency. Together, this preliminary evidence suggests that regions of anterior prefrontal, parietal and temporal cortex are involved in lucid dreaming. The involvement of these brain regions in metacognitive processes during the waking state is also in line with these findings.
A primary goal is to develop reliable strategies for making lucid dreaming more accessible. As reviewed above, several studies have explored methods for non-invasive electrical stimulation of the brain as well as pharmacological approaches to lucid dream induction. Electrical stimulation of prefrontal brain areas has resulted in statistically significant but weak increases of dream “insight” ratings, but so far it has not resulted in significant increases in the frequency of lucid dreams. Currently it remains too early to tell how effective (if at all) electrical stimulation of the frontal cortex, or other brain areas, could be for lucid dream induction. Pharmacological induction with agents acting on the cholinergic system, in particular the AChEI galantamine, has shown promising results; however, these findings need to be replicated systematically, and lucid dreams objectively confirmed with polysomnography. Other approaches to lucid dream induction not discussed in the current review, and that do not directly target neural mechanisms, such as cognitive/psychological approaches, also appear promising ( Stumbrys et al., 2012 ). For example, advances in the research of targeted memory reactivation via olfactory or acoustic stimuli during sleep (e.g., Oudiette, Antony, Creery and Paller, 2013 ) might lead to new strategies for lucid dream induction, together with continued research on stimulating lucid dreams with visual or auditory cues ( LaBerge and Levitan, 1995 ; LaBerge et al., 1988 ; LaBerge et al., 1981b ).
In conclusion, additional studies with larger sample sizes, for example large-scale group-level high-density EEG, MEG, and concurrent EEG/fMRI studies, will be important next steps toward characterizing the neural functional changes associated with lucid dreaming. For now, a more detailed understanding of the neurobiological basis of lucid dreaming remains an open question for ongoing research. Lucid dreaming shows promise as a useful methodology for psychophysiological studies of REM sleep, with potential applications in both clinical and basic research domains. Perhaps the largest potential of research on lucid dreaming is that it provides a unique method to investigate the neurobiology of consciousness, which remains one of the largest lacunas in scientific knowledge.
- EEG studies of lucid dreaming are mostly underpowered and show mixed results
- Preliminary neuroimaging data implicates frontoparietal cortices in lucid dreaming
- Cholinergic stimulation with mental set shows promise for inducing lucid dreams
- We present best-practice procedures to investigate lucid dreaming in the laboratory
Acknowledgements
We thank Stephen LaBerge for helpful discussion.
BB was supported by the National Institutes of Health (NIH) under Ruth L. Kirschstein National Research Service Award F32NS089348 from the NINDS. SAM-R was supported by the Coordena9ao de Aperfei9oamento de Pessoal de Nivel Superior (CAPES), Conselho Nacional de Desenvolvimento Científico e Tecnológico (CNPq), Financiadora de Estudos e Projetos do Ministério da Ciência e Tecnologia (FINEP), and Fundação de Apoio à Pesquisa do Estado do Rio Grande do Norte (FAPERN). MD was supported by the Netherlands Organisation for Scientific Research (NWO) through a Vidi fellowship (016.Vidi.185.142). The content is solely the responsibility of the authors and does not necessarily represent the official views of the NIH, CAPES, CNPq, FINEP, FAPERN or NWO.
Publisher's Disclaimer: This is a PDF file of an unedited manuscript that has been accepted for publication. As a service to our customers we are providing this early version of the manuscript. The manuscript will undergo copyediting, typesetting, and review of the resulting proof before it is published in its final citable form. Please note that during the production process errors may be discovered which could affect the content, and all legal disclaimers that apply to the journal pertain.
Competing interests
The authors declare no competing interests.
- Abramovitch H, 1995. The nightmare of returning home: A case of acute onset nightmare disorder treated by lucid dreaming . Israel journal of psychiatry and related sciences 32 , 140–145. [ PubMed ] [ Google Scholar ]
- Amatruda TT, Black DA, McKenna TM, McCarley RW, Hobson JA, 1975. Sleep cycle control and cholinergic mechanisms: Differential effects of carbachol injections at pontine brain stem sites . Brain Res . 98 , 501–515. [ PubMed ] [ Google Scholar ]
- Antrobus JS, Antrobus JS, 1967. Discrimination of two sleep stages by human subjects . Psychophysiology 4 , 48–55. [ PubMed ] [ Google Scholar ]
- Aristotle, 1941. On Dreams, in: McKeon R (Ed.), The basic works of Aristotle . Random House, New York, NY, pp. 618–625. [ Google Scholar ]
- Arnulf I, 2011. The’scanning hypothesis’ of rapid eye movements during REM sleep: A review of the evidence . Arch. Ital. Biol 149 , 367–382. [ PubMed ] [ Google Scholar ]
- Aspy DJ, Delfabbro P, Proeve M, Mohr P, 2017. Reality testing and the Mnemonic Induction of Lucid Dreams: Findings from the national Australian lucid dream induction study . Dreaming 27 , 206–231. [ Google Scholar ]
- Aviram L, Soffer-Dudek N, 2018. Lucid dreaming: Intensity, but not frequency, is inversely related to psychopathology . Front. Psychol 9 , 384. [ PMC free article ] [ PubMed ] [ Google Scholar ]
- Baghdoyan HA, 1997. Cholinergic mechanisms regulating REM sleep, in: Schwartz W (Ed.), Sleep science: Integrating basic research and clinical practice. Karger , Basel, pp. 88–116. [ Google Scholar ]
- Baier M, 2010. Insight in schizophrenia: A review . Curr. Psychiatry Rep 12 , 356–361. [ PubMed ] [ Google Scholar ]
- Baird B, Castelnovo A, Gosseries O, Tononi G, 2018a. Frequent lucid dreaming associated with increased functional connectivity between frontopolar cortex and temporoparietal association areas . Sci. Rep [ PMC free article ] [ PubMed ] [ Google Scholar ]
- Baird B, Castelnovo A, Riedner BA, Lutz A, Ferrarelli F, Boly M, Davidson RJ, Tononi G, 2018b. Human rapid eye movement sleep shows local increases in low-frequency oscillations and global decreases in high-frequency oscillations compared to resting wakefulness . eNeuro 5. [ PMC free article ] [ PubMed ] [ Google Scholar ]
- Baird B, Riedner BA, Boly M, Davidson RJ, Tononi G, 2018c. Increased lucid dream frequency in long-term meditators but not following mindfulness-based stress reduction training. Psychology of Consciousness: Theory , Research and Practice . [ PMC free article ] [ PubMed ] [ Google Scholar ]
- Baird B, Smallwood J, Gorgolewski KJ, Margulies DS, 2013. Medial and lateral networks in anterior prefrontal cortex support metacognitive ability for memory and perception . J. Neurosci 33 , 16657–16665. [ PMC free article ] [ PubMed ] [ Google Scholar ]
- Bentley P, Driver J, Dolan RJ, 2011. Cholinergic modulation of cognition: Insights from human pharmacological functional neuroimaging . Prog. Neurobiol 94 , 360–388. [ PMC free article ] [ PubMed ] [ Google Scholar ]
- Berrios GE, Markova IS, 1998. Insight in the psychoses: A conceptual history, in: Amador X, David A (Eds.), Insight and psychosis . Oxford University Press, New York, NY, pp. 33–46. [ Google Scholar ]
- Berryhill ME, Phuong L, Picasso L, Cabeza R, Olson IR, 2007. Parietal lobe and episodic memory: Bilateral damage causes impaired free recall of autobiographical memory . J. Neurosci 27 , 14415–14423. [ PMC free article ] [ PubMed ] [ Google Scholar ]
- Blumenfeld H, 2008. Epilepsy and consciousness, in: Laureys S (Ed.), The neurology of consciousness . Elsevier Academic Press, Oxford, pp. 247–260. [ Google Scholar ]
- Boly M, Garrido MI, Gosseries O, Bruno M-A, Boveroux P, Schnakers C, Massimini M, Litvak V, Laureys S, Friston K, 2011. Preserved feedforward but impaired top-down processes in the vegetative state . Science 332 , 858–862. [ PubMed ] [ Google Scholar ]
- Boly M, Moran R, Murphy M, Boveroux P, Bruno M-A, Noirhomme Q, Ledoux D, Bonhomme V, Brichant J-F, Tononi G, 2012. Connectivity changes underlying spectral EEG changes during propofol-induced loss of consciousness . J. Neurosci 32 , 7082–7090. [ PMC free article ] [ PubMed ] [ Google Scholar ]
- Braun A, Balkin T, Wesenten N, Carson R, Varga M, Baldwin P, Selbie S, Belenky G, Herscovitch P, 1997. Regional cerebral blood flow throughout the sleep-wake cycle. An H2 (15) O PET study . Brain 120 , 1173–1197. [ PubMed ] [ Google Scholar ]
- Braun AR, Balkin TJ, Wesensten NJ, Gwadry F, Carson RE, Varga M, Baldwin P, Belenky G, Herscovitch P, 1998. Dissociated pattern of activity in visual cortices and their projections during human rapid eye movement sleep . Science 279 , 91–95. [ PubMed ] [ Google Scholar ]
- Brylowski A, Levitan L, LaBerge S, 1989. H-reflex suppression and autonomic activation during lucid REM sleep: A case study . Sleep 12 , 374–378. [ PubMed ] [ Google Scholar ]
- Cavallero C, Cicogna P, Natale V, Occhionero M, Zito A, 1992. Slow wave sleep dreaming . Sleep 15 , 562–566. [ PubMed ] [ Google Scholar ]
- Cavanna AE, Trimble MR, 2006. The precuneus: A review of its functional anatomy and behavioural correlates . Brain 129 , 564–583. [ PubMed ] [ Google Scholar ]
- Christoff K, Ream JM, Geddes L, Gabrieli JD, 2003. Evaluating self-generated information: Anterior prefrontal contributions to human cognition . Behav. Neurosci 117 , 1161–1168. [ PubMed ] [ Google Scholar ]
- Cuadra G, Summers K, Giacobini E, 1994. Cholinesterase inhibitor effects on neurotransmitters in rat cortex in vivo . J. Pharmacol. Exp. Ther 270 , 277–284. [ PubMed ] [ Google Scholar ]
- Dane J, Van de Caslte R, 1984. A comparison of waking instruction and posthypnotic suggestion for lucid dream induction . Lucidity Letter 3. [ Google Scholar ]
- David AS, 1990. Insight and psychosis . The British Journal of Psychiatry 156 , 798–808. [ PubMed ] [ Google Scholar ]
- DeGracia DJ, LaBerge S, 2000. Varieties of lucid dreaming experience, in: Kunzendorf RG, Wallace B (Eds.), Individual differences in conscious experience . John Benjamins, Amsterdam, pp. 269–307. [ Google Scholar ]
- Dement W, Wolpert EA, 1958. The relation of eye movements, body motility, and external stimuli to dream content . J. Exp. Psychol 55 , 543–552. [ PubMed ] [ Google Scholar ]
- Dixon ML, De La Vega A, Mills C, Andrews-Hanna J, Spreng RN, Cole MW, Christoff K, 2018. Heterogeneity within the frontoparietal control network and its relationship to the default and dorsal attention networks . Proc. Natl. Acad. Sci , E1598–E1607. [ PMC free article ] [ PubMed ] [ Google Scholar ]
- Dodet P, Chavez M, Leu-Semenescu S, Golmard J, Arnulf I, 2014. Lucid dreaming in narcolepsy . Sleep 38 , 487–497. [ PMC free article ] [ PubMed ] [ Google Scholar ]
- Donner TH, Siegel M, 2011. A framework for local cortical oscillation patterns . Trends Cog. Sci 15 , 191–199. [ PubMed ] [ Google Scholar ]
- Dresler M, Eibl L, Fischer CF, Wehrle R, Spoormaker VI, Steiger A, Czisch M, Pawlowski M, 2014. Volitional components of consciousness vary across wakefulness, dreaming and lucid dreaming . Front. Psychol 4 , 987. [ PMC free article ] [ PubMed ] [ Google Scholar ]
- Dresler M, Koch SP, Wehrle R, Spoormaker VI, Holsboer F, Steiger A, Sämann PG, Obrig H, Czisch M, 2011. Dreamed movement elicits activation in the sensorimotor cortex . Curr. Biol 21 , 1833–1837. [ PubMed ] [ Google Scholar ]
- Dresler M, Sandberg A, Bublitz C, Ohla K, Trenado C, Mroczko-Wqsowicz A, Kühn S, Repantis D, 2018. Hacking the brain: Dimensions of cognitive enhancement . ACS Chemical Neuroscience . [ PMC free article ] [ PubMed ] [ Google Scholar ]
- Dresler M, Wehrle R, Spoormaker VI, Koch SP, Holsboer F, Steiger A, Obrig H, Sämann PG, Czisch M, 2012. Neural correlates of dream lucidity obtained from contrasting lucid versus non-lucid REM sleep: A combined EEG/fMRI case study . Sleep 35 , 1017–1020. [ PMC free article ] [ PubMed ] [ Google Scholar ]
- Dresler M, Wehrle R, Spoormaker VI, Steiger A, Holsboer F, Czisch M, Hobson JA, 2015. Neural correlates of insight in dreaming and psychosis . Sleep Med. Rev 20 , 92–99. [ PubMed ] [ Google Scholar ]
- Engel AK, Fries P, 2010. Beta-band oscillations—signalling the status quo? Curr. Opin. Neurobiol 20 , 156–165. [ PubMed ] [ Google Scholar ]
- Engel J, Kuhl DE, Phelps ME, 1982. Patterns of human local cerebral glucose metabolism during epileptic seizures . Science 218 , 64–66. [ PubMed ] [ Google Scholar ]
- Erlacher D, Schredl M, LaBerge S, 2003. Motor area activation during dreamed hand clenching: A pilot study on EEG alpha band . Sleep Hypnosis 5 , 182–187. [ Google Scholar ]
- Filevich E, Dresler M, Brick TR, Kühn S, 2015. Metacognitive mechanisms underlying lucid dreaming . J. Neurosci 35 , 1082–1088. [ PMC free article ] [ PubMed ] [ Google Scholar ]
- Fleming SM, Weil RS, Nagy Z, Dolan RJ, Rees G, 2010. Relating introspective accuracy to individual differences in brain structure . Science 329 , 1541–1543. [ PMC free article ] [ PubMed ] [ Google Scholar ]
- Fosse R, 2000. REM mentation in narcoleptics and normals: An empirical test of two neurocognitive theories . Conscious. Cogn 9 , 488–509. [ PubMed ] [ Google Scholar ]
- Funk CM, Honjoh S, Rodriguez AV, Cirelli C, Tononi G, 2016. Local slow waves in superficial layers of primary cortical areas during REM sleep . Curr. Biol 26 , 396–403. [ PMC free article ] [ PubMed ] [ Google Scholar ]
- Gackenbach J, Cranson R, Alexander C, 1986. Lucid dreaming, witnessing dreaming, and the transcendental meditation technique: A developmental relationship . Lucidity Letter 5 , 34–40. [ Google Scholar ]
- Gackenbach J, LaBerge S, 1988. Conscious mind, sleeping brain: Perspectives on lucid dreaming . Plenum Press, New York, NY. [ Google Scholar ]
- Garfield P, Fellows P, Halliday G, Malamud JR, 1988. Clinical applications of lucid dreaming, in: Gackenbach J, LaBerge S (Eds.), Conscious Mind, Sleeping Brain . Plenum Press, New York, NY, pp. 289–319. [ Google Scholar ]
- Giacobini E, Zhu X-D, Williams E, Sherman K, 1996. The effect of the selective reversible acetylcholinesterase inhibitor E2020 on extracellular acetylcholine and biogenic amine levels in rat cortex . Neuropharmacology 35 , 205–211. [ PubMed ] [ Google Scholar ]
- Gillin JC, Sitaram N, Janowsky D, Risch C, Huey L, Storch FI, 1985. Cholinergic mechanisms in REM sleep, in: Wauquier A, Gail lard J, Monti J, Radulovacki M (Eds.), Sleep: Neurotransmitters and neuromodulators . Raven Press, New York, NY, pp. 29–42. [ Google Scholar ]
- Goldberg G, 1985. Supplementary motor area structure and function: Review and hypotheses. Behav . Brain. Sci 8 , 567–588. [ Google Scholar ]
- Gratton G, Coles MG, Donchin E, 1983. A new method for off-line removal of ocular artifact . Electroencephalogr. Clin. Neurophysiol 55 , 468–484. [ PubMed ] [ Google Scholar ]
- Green CE, 1968. Lucid dreams. Hamilton , London. [ Google Scholar ]
- Hallett M, 2007. Transcranial magnetic stimulation: A primer . Neuron 55 , 187–199. [ PubMed ] [ Google Scholar ]
- Hearne KM, 1978. Lucid dreams: An elecro-physiological and psychological study (Unpublished doctoral dissertation) . Liverpool University, Liverpool, UK. [ Google Scholar ]
- Hermann DM, Siccoli M, Brugger P, Wachter K, Mathis J, Achermann P, Bassetti CL, 2008. Evolution of neurological, neuropsychological and sleep-wake disturbances after paramedian thalamic stroke . Stroke 39 , 62–68. [ PubMed ] [ Google Scholar ]
- Hernandez-Peon R, Chavez-lbarra G, Morgane P, Timo-Iaria C, 1963. Limbic cholinergic pathways involved in sleep and emotional behavior . Exp. Neurol 8 , 93–111. [ Google Scholar ]
- Hipp JF, Siegel M, 2013. Dissociating neuronal gamma-band activity from cranial and ocular muscle activity in EEG . Front. Hum. Neurosci 7 , 338. [ PMC free article ] [ PubMed ] [ Google Scholar ]
- Hobson JA, Pace-Schott EF, 2002. The cognitive neuroscience of sleep: Neuronal systems, consciousness and learning . Nat. Rev. Neurosci 3 , 679–693. [ PubMed ] [ Google Scholar ]
- Hobson JA, Pace-Schott EF, Stickgold R, 2000. Dreaming and the brain: Toward a cognitive neuroscience of conscious states . Behav. Brain. Sci 23 , 793–842. [ PubMed ] [ Google Scholar ]
- Hodes R, Dement WC, 1964. Depression of electrically induced reflexes (“H-reflexes”) in man during low voltage EEG “sleep” . Electroencephalogr. Clin. Neurophysiol 17 , 617–629. [ PubMed ] [ Google Scholar ]
- Holzinger B, Klösch G, Saletu B, 2015. Studies with lucid dreaming as add-on therapy to Gestalt therapy . Acta Neurol. Scand 131 , 355–363. [ PubMed ] [ Google Scholar ]
- Holzinger B, LaBerge S, Levitan L, 2006. Psychophysiological correlates of lucid dreaming . Dreaming 16 , 88–95. [ Google Scholar ]
- Joseph R, 1999. Frontal lobe psychopathology: Mania, depression, confabulation, catatonia, perseveration, obsessive compulsions, and schizophrenia . Psychiatry 62 , 138–172. [ PubMed ] [ Google Scholar ]
- Kahan TL, LaBerge S, Levitan L, Zimbardo P, 1997. Similarities and differences between dreaming and waking cognition: An exploratory study . Conscious. Cogn 6 , 132–147. [ PubMed ] [ Google Scholar ]
- Kahan TL, Sullivan KT, 2012. Assessing metacognitive skills in waking and sleep: A psychometric analysis of the Metacognitive, Affective, Cognitive Experience (MACE) questionnaire . Conscious. Cogn 21 , 340–352. [ PubMed ] [ Google Scholar ]
- Keren AS, Yuval-Greenberg S, Deouell LY, 2010. Saccadic spike potentials in gamma-band EEG: Characterization, detection and suppression . Neuroimage 49 , 2248–2263. [ PubMed ] [ Google Scholar ]
- Kern S, Appel K, Schredl M, Pipa G, 2017. No effect of α-GPC on lucid dream induction or dream content . Somnologie 21 , 180–186. [ Google Scholar ]
- Kjaer TW, Nowak M, Lou HC, 2002. Reflective self-awareness and conscious states: PET evidence for a common midline parietofrontal core . Neuroimage 17 , 1080–1086. [ PubMed ] [ Google Scholar ]
- Komogortsev OV, Karpov A, 2013. Automated classification and scoring of smooth pursuit eye movements in the presence of fixations and saccades . Behav. Res. Methods 45 , 203–215. [ PubMed ] [ Google Scholar ]
- Koontz J, Baskys A, 2005. Effects of galantamine on working memory and global functioning in patients with mild cognitive impairment: A double-blind placebo-controlled study . Am. J. Alzheimer’s Dis. Other Dementias 20 , 295–302. [ PMC free article ] [ PubMed ] [ Google Scholar ]
- Koshino Y, Niedermeyer E, 1975. Enhancement of rolandic mu-rhythm by pattern vision . Electroencephalogr. Clin. Neurophysiol 38 , 535–538. [ PubMed ] [ Google Scholar ]
- LaBerge S, 1980a. Lucid dreaming as a learnable skill: A case study . Percept. Motor Skills 51 , 1039–1042. [ Google Scholar ]
- LaBerge S, 1980b. Lucid dreaming: An exploratory study of consciousness during sleep (Unpublished doctoral dissertation) . Stanford University, Stanford, CA. [ Google Scholar ]
- LaBerge S, 1985. Lucid dreaming: The power of being awake and aware in your dreams . Jeremy P. Tarcher, Los Angeles. [ Google Scholar ]
- LaBerge S, 1988a. Lucid dreaming in western literature, in: LaBerge JGS (Ed.), Conscious Mind, Sleeping Brain . Plenum Press, New York, NY, pp. 11–26. [ Google Scholar ]
- LaBerge S, 1988b. The psychophysiology of lucid dreaming, in: Gackenbach J, LaBerge S (Eds.), Conscious mind, sleeping brain: Perspectives on lucid dreaming . Plenum Press, New York, NY, pp. 135–153. [ Google Scholar ]
- LaBerge S, 1990. Lucid dreaming: Psychophysiological studies of consciousness during REM sleep, in: Bootzin RR, Kihlstrom JF, Schacter DL (Eds.), Sleep and Cognition . American Psychological Association, Washington DC, pp. 109–126. [ Google Scholar ]
- LaBerge S, 2001. The paradox and promise of lucid dreaming Research update: Cholinergic stimulation of lucid dreaming; voluntary control of auditory perception during REM lucid dreams, International Association for the Study of Dreams , Berkeley. [ Google Scholar ]
- LaBerge S, 2003. Lucid dreaming and the yoga of the dream state: A psychophysiological perspective, in: Wallace BA (Ed.), Buddhism & science: Breaking new ground . Columbia University Press, New York, NY, pp. 233–258. [ Google Scholar ]
- LaBerge S, 2010. Signal-verfied lucid dreaming proves that REM sleep can support reflective consciousness . Int. J. Dream Res 3 , 26–27. [ Google Scholar ]
- LaBerge S, 2015. Lucid dreaming: Metaconsciousness during paradoxical sleep, in: Glucksman MKM (Ed.), Dream research: Contributions to clinical practice . Routledge, New York, NY, pp. 198–214. [ Google Scholar ]
- LaBerge S, Baird B, Zimbardo PG, 2018a. Smooth tracking of visual targets distinguishes lucid REM sleep dreaming and waking perception from imagination . Nat. Comm 9 , 3298. [ PMC free article ] [ PubMed ] [ Google Scholar ]
- LaBerge S, Dement WC, 1982. Voluntary control of respiration during REM sleep . Sleep Res . 11 , 107. [ Google Scholar ]
- LaBerge S, Greenleaf W, Kedzierski B, 1983. Physiological responses to dreamed sexual activity during lucid REM sleep . Psychophysiology , 454–455. [ Google Scholar ]
- LaBerge S, LaMarca K, Baird B, 2018b. Pre-sleep treatment with galantamine stimulates lucid dreaming: A double-blind, placebo-controlled, crossover study . PLoS ONE 13 , e0201246. [ PMC free article ] [ PubMed ] [ Google Scholar ]
- LaBerge S, Levitan L, 1995. Validity established of DreamLight cues for eliciting lucid dreaming . Dreaming 5 , 159–168. [ Google Scholar ]
- LaBerge S, Levitan L, Dement WC, 1986. Lucid dreaming: Physiological correlates of consciousness during REM sleep . J. Mind Behav 7 , 251–258. [ Google Scholar ]
- LaBerge S, Levitan L, Rich R, Dement WC, 1988. Induction of lucid dreaming by light stimulation during REM sleep . Sleep Res . 17 , 104. [ Google Scholar ]
- LaBerge S, Nagel L, Taylor W, Dement W, Zarcone V, 1981a. Psychophysiological correlates of the initiation of lucid dreaming . Sleep Res . 10 , 149. [ Google Scholar ]
- LaBerge S, Owens J, Nagel L, Dement WC, 1981b. “This is a dream”: Induction of lucid dreams by verbal suggestion during REM sleep . Sleep Res . 10 , 150. [ Google Scholar ]
- LaBerge S, Phillips L, Levitan L, 1994. An hour of wakefulness before morning naps makes lucidity more likely . NightLight 6 , 1–4. [ Google Scholar ]
- LaBerge S, Rheingold H, 1990. Exploring the world of lucid dreaming . Ballantine Books; New York, NY. [ Google Scholar ]
- LaBerge SP, Nagel LE, Dement WC, Zarcone VP, 1981c. Lucid dreaming verified by volitional communication during REM sleep . Percept. Motor Skills 52 , 727–732. [ PubMed ] [ Google Scholar ]
- Lachaux JP, Fonlupt P, Kahane P, Minotti L, Hoffmann D, Bertrand O, Baciu M, 2007. Relationship between task-related gamma oscillations and BOLD signal: New insights from combined fMRI and intracranial EEG . Hum. Brain Mapp 28 , 1368–1375. [ PMC free article ] [ PubMed ] [ Google Scholar ]
- Lancee J, Van Den Bout J, Spoormaker VI, 2010. Expanding self-help imagery rehearsal therapy for nightmares with sleep hygiene and lucid dreaming: A waiting-list controlled trial . Int. J. Dream Res 3 , 111–120. [ Google Scholar ]
- Laureys S, Owen AM, Schiff ND, 2004. Brain function in coma, vegetative state, and related disorders . Lancet Neurol . 3 , 537–546. [ PubMed ] [ Google Scholar ]
- Laureys S, Perrin F, Brédart S, 2007. Self-consciousness in non-communicative patients . Conscious. Cogn 16 , 722–741. [ PubMed ] [ Google Scholar ]
- Lee U, Ku S, Noh G, Baek S, Choi B, Mashour GA, 2013. Disruption of frontal-parietal communication by ketamine, propofol, and sevoflurane . Anesthesiology 118 , 1264–1275. [ PMC free article ] [ PubMed ] [ Google Scholar ]
- Levin R, Nielsen TA, 2007. Disturbed dreaming, posttraumatic stress disorder, and affect distress: A review and neurocognitive model . Psychol. Bull 133 , 482. [ PubMed ] [ Google Scholar ]
- Lincoln TM, Lüllmann E, Rief W, 2007. Correlates and long-term consequences of poor insight in patients with schizophrenia. A systematic review . Schizophrenia Bulletin 33 , 1324–1342. [ PMC free article ] [ PubMed ] [ Google Scholar ]
- Lou HC, Luber B, Crupain M, Keenan JP, Nowak M, Kjaer TW, Sackeim HA, Lisanby SH, 2004. Parietal cortex and representation of the mental self . Proc. Natl. Acad. Sci 101 , 6827–6832. [ PMC free article ] [ PubMed ] [ Google Scholar ]
- Luigetti M, Di Lazzaro V, Broccolini A, Vollono C, Dittoni S, Frisullo G, Pilato F, Profice P, Losurdo A, Morosetti R, 2011. Bilateral thalamic stroke transiently reduces arousals and NREM sleep instability . J. Neurol. Sci 300 , 151–154. [ PubMed ] [ Google Scholar ]
- Malcolm N, 1959. Dreaming . Routledge, London, England. [ Google Scholar ]
- Maquet P, Péters J-M, Aerts J, Delfiore G, Degueldre C, Luxen A, Franck G, 1996. Functional neuroanatomy of human rapid-eye-movement sleep and dreaming . Nature 383 , 163–166. [ PubMed ] [ Google Scholar ]
- Mashour GA, 2014. Top-down mechanisms of anesthetic-induced unconsciousness . Front. Sys. Neurosci 8 , 115. [ PMC free article ] [ PubMed ] [ Google Scholar ]
- Mason LI, Orme-Johnson DW, 2010. Transcendental consciousness wakes up in dreaming and deep sleep . Int. J. Dream Res 3 , 28–32. [ Google Scholar ]
- Mazzetti M, Bellucci C, Mattarozzi K, Plazzi G, Tuozzi G, Cipolli C, 2010. REM-dreams recall in patients with narcolepsy-cataplexy . Brain Res. Bull 81 , 133–140. [ PubMed ] [ Google Scholar ]
- McCaig RG, Dixon M, Keramatian K, Liu I, Christoff K, 2011. Improved modulation of rostrolateral prefrontal cortex using real-time fMRI training and meta-cognitive awareness . NeuroImage 55 , 1298–1305. [ PubMed ] [ Google Scholar ]
- McKay LC, Adams L, Frackowiak RS, Corfield DR, 2008. A bilateral cortico-bulbar network associated with breath holding in humans, determined by functional magnetic resonance imaging . NeuroImage 40 , 1824–1832. [ PubMed ] [ Google Scholar ]
- Mintz AR, Dobson KS, Romney DM, 2003. Insight in schizophrenia: A meta-analysis . Schizophrenia Research 61 , 75–88. [ PubMed ] [ Google Scholar ]
- Montagna P, 2005. Fatal familial insomnia: A model disease in sleep physiopathology . Sleep Med. Rev 9 , 339–353. [ PubMed ] [ Google Scholar ]
- Morgenthaler TI, Auerbach S, Casey KR, Kristo D, Maganti R, Ramar K, Zak R, Kartje R, 2018. Position paper for the treatment of nightmare disorder in adults: An American Academy of Sleep Medicine position paper . Journal of Clinical Sleep Medicine 14 , 1041–1055. [ PMC free article ] [ PubMed ] [ Google Scholar ]
- Mota NB, Resende A, Mota-Rolim SA, Copelli M, Ribeiro S, 2016. Psychosis and the control of lucid dreaming . Front. Psychol 7 , 294. [ PMC free article ] [ PubMed ] [ Google Scholar ]
- Mota-Rolim S, Pantoja A, Pinheiro R, Camilo A, Barbosa T, Hazboun I, Araujo J, Ribeiro S, 2008. Lucid dream: Sleep electroencephalographic features and behavioral induction methods, First Congress IBRO/LARC of Neurosciences for Latin America, Caribbean and Iberian Peninsula . Bdzios, Brazil. [ Google Scholar ]
- Mota-Rolim SA, Araujo JF, 2013. Neurobiology and clinical implications of lucid dreaming . Medical hypotheses 81 , 751–756. [ PubMed ] [ Google Scholar ]
- Mota-Rolim SA, Brandão DS, Andrade KC, de Queiroz CMT, Araujo JF, de Araujo DB, Ribeiro S, 2015. Neurophysiological features of lucid dreaming during N1 and N2 sleep stages: Two case reports . Sleep Sci . 4 , 215. [ Google Scholar ]
- Mota-Rolim SA, Erlacher D, Tort AB, Araujo JF, Ribeiro S, 2010. Different kinds of subjective experience during lucid dreaming may have different neural sub-strates . Int. J. Dream Res 25 , 550–557. [ Google Scholar ]
- Mota-Rolim SA, Targino ZH, Souza BC, Blanco W, Araujo JF, Ribeiro S, 2013. Dream characteristics in a Brazilian sample: An online survey focusing on lucid dreaming . Front. Hum. Neurosci 7 , 836. [ PMC free article ] [ PubMed ] [ Google Scholar ]
- Muzio JN, Roffwarg HP, Kaufman E, 1966. Alterations in the nocturnal sleep cycle resulting from LSD . Clin. Neurophysiol 21 , 313–324. [ PubMed ] [ Google Scholar ]
- Nieminen JO, Gosseries O, Massimini M, Saad E, Sheldon AD, Boly M, Siclari F, Postle BR, Tononi G, 2016. Consciousness and cortical responsiveness: A within-state study during non-rapid eye movement sleep . Sci. Rep 6 , 30932. [ PMC free article ] [ PubMed ] [ Google Scholar ]
- Nir Y, Fisch L, Mukamel R, Gelbard-Sagiv H, Arieli A, Fried I, Malach R, 2007. Coupling between neuronal firing rate, gamma LFP, and BOLD fMRI is related to interneuronal correlations . Curr. Biol 17 , 1275–1285. [ PubMed ] [ Google Scholar ]
- Nir Y, Tononi G, 2010. Dreaming and the brain: From phenomenology to neurophysiology . Trends Cog. Sci 14 , 88–100. [ PMC free article ] [ PubMed ] [ Google Scholar ]
- Norbu N, Katz M, 1992. Dream yoga and the practice of natural light . Snow Lion Publications, Ithaca, NY. [ Google Scholar ]
- Noreika V, Windt JM, Lenggenhager B, Karim AA, 2010. New perspectives for the study of lucid dreaming: From brain stimulation to philosophical theories of self-consciousness . Int. J. Dream Res 3 , 36–45. [ Google Scholar ]
- Ogilvie R, Hunt H, Kushniruk A, Newman J, 1983. Lucid dreams and the arousal continuum . Lucidity Letter 2. [ Google Scholar ]
- Ogilvie R, Hunt H, Sawicki C, McGowan K, 1978. Searching for lucid dreams . Sleep Res . 7 , 165. [ Google Scholar ]
- Ogilvie R, Vieira K, Small R, 1991. EEG activity during lucid dreaming . Lucidity Letter 10. [ Google Scholar ]
- Ogilvie RD, Hunt HT, Tyson PD, Lucescu ML, Jeakins DB, 1982. Lucid dreaming and alpha activity: A preliminary report . Percept. Motor Skills 55 , 795–808. [ PubMed ] [ Google Scholar ]
- Oudiette D, Antony JW, Creery JD, Paller KA, 2013. The role of memory reactivation during wakefulness and sleep in determining which memories endure . J. Neurosci 33 , 6672–6678. [ PMC free article ] [ PubMed ] [ Google Scholar ]
- Oudiette D, Dodet P, Ledard N, Artru E, Rachidi I, Similowski T, Arnulf I, 2018. REM sleep respiratory behaviours mental content in narcoleptic lucid dreamers . Sci. Rep 8 , 2636. [ PMC free article ] [ PubMed ] [ Google Scholar ]
- Padmasambhava, 1998. Natural liberation: Padmasambhava’s teachings on the six bardos . Wisdom, Boston, MA. [ Google Scholar ]
- Perogamvros L, Baird B, Seibold M, Riedner B, Boly M, Tononi G, 2017. The phenomenal contents and neural correlates of spontaneous thoughts across wakefulness, NREM sleep, and REM sleep . J. Cogn. Neurosci 29 , 1766–1777. [ PMC free article ] [ PubMed ] [ Google Scholar ]
- Pfurtscheller G, Stancak A, Neuper C, 1996. Post-movement beta synchronization. A correlate of an idling motor area? Electroencephalogr. Clin. Neurophysiol 98 , 281–293. [ PubMed ] [ Google Scholar ]
- Pigorini A, Sarasso S, Proserpio P, Szymanski C, Arnulfo G, Casarotto S, Fecchio M, Rosanova M, Mariotti M, Russo GL, 2015. Bistability breaks-off deterministic responses to intracortical stimulation during non-REM sleep . Neuroimage 112 , 105–113. [ PubMed ] [ Google Scholar ]
- Price RF, Cohen DB, 1988. Lucid dream induction: An empirical evaluation . Conscious mind, sleeping brain: Perspectives on lucid dreaming , 105–134. [ Google Scholar ]
- Purdon PL, Pierce ET, Mukamel EA, Prerau MJ, Walsh JL, Wong KFK, Salazar-Gomez AF, Harrell PG, Sampson AL, Cimenser A, 2013. Electroencephalogram signatures of loss and recovery of consciousness from propofol . Proc. Natl. Acad. Sci 110 , E1142–E1151. [ PMC free article ] [ PubMed ] [ Google Scholar ]
- Raggi A, Cosentino FI, Lanuzza B, Ferri R, 2010. Behavioural and neurophysiologic features of state dissociation: A brief review of the literature and three descriptive case studies . Behavioural neurology 22 , 91–99. [ PMC free article ] [ PubMed ] [ Google Scholar ]
- Rak M, Beitinger P, Steiger A, Schredl M, Dresler M, 2015. Increased lucid dreaming frequency in narcolepsy . Sleep 38 , 787–792. [ PMC free article ] [ PubMed ] [ Google Scholar ]
- Rechtschaffen A, Kales A, 1968. A manual of standardized terminology, techniques and scoring system for sleep stages of human subjects . Government Printing Office National Institutes of Health, Washington, DC. [ PubMed ] [ Google Scholar ]
- Rees G, Wojciulik E, Clarke K, Husain M, Frith C, Driver J, 2002. Neural correlates of conscious and unconscious vision in parietal extinction . Neurocase 8 , 387–393. [ PubMed ] [ Google Scholar ]
- Riemann D, Gann H, Dressing H, Müller WE, Aldenhoff JB, 1994. Influence of the cholinesterase inhibitor galanthamine hydrobromide on normal sleep . Psychiatry Res . 51 , 253–267. [ PubMed ] [ Google Scholar ]
- Roehrs T, Roth T, 2001. Sleep, sleepiness, sleep disorders and alcohol use and abuse . Sleep Med. Rev 5 , 287–297. [ PubMed ] [ Google Scholar ]
- Sagnier S, Coulon P, Chaufton C, Poll M, Debruxelles S, Renou P, Rouanet F, Olindo S, Sibon I, 2015. Lucid dreams, an atypical sleep disturbance in anterior and mediodorsal thalamic strokes . Revue neurologique 171 , 768–772. [ PubMed ] [ Google Scholar ]
- Saunders DT, Roe CA, Smith G, Clegg H, 2016. Lucid dreaming incidence: A quality effects meta-analysis of 50 years of research . Conscious. Cogn 43 , 197–215. [ PubMed ] [ Google Scholar ]
- Schierenbeck T, Riemann D, Berger M, Hornyak M, 2008. Effect of illicit recreational drugs upon sleep: Cocaine, ecstasy and marijuana . Sleep Med. Rev 12 , 381–389. [ PubMed ] [ Google Scholar ]
- Schmitz TW, Rowley HA, Kawahara TN, Johnson SC, 2006. Neural correlates of self-evaluative accuracy after traumatic brain injury . Neuropsychologia 44 , 762–773. [ PubMed ] [ Google Scholar ]
- Schnakers C, Vanhaudenhuyse A, Giacino J, Ventura M, Boly M, Majerus S, Moonen G, Laureys S, 2009. Diagnostic accuracy of the vegetative and minimally conscious state: Clinical consensus versus standardized neurobehavioral assessment . BMC Neurol . 9 , 35. [ PMC free article ] [ PubMed ] [ Google Scholar ]
- Schooler JW, 2002. Re-representing consciousness: Dissociations between experience and meta-consciousness . Trends Cog. Sci 6 , 339–344. [ PubMed ] [ Google Scholar ]
- Schredl M, Erlacher D, 2004. Lucid dreaming frequency and personality . Pers. Individ. Dif 37 , 1463–1473. [ Google Scholar ]
- Schredl M, Rieger J, Göritz AS, 2018. Measuring lucid dreaming skills: A new questionnaire (LUSK) . Int. J. Dream Res [ Google Scholar ]
- Siclari F, Baird B, Perogamvros L, Bernardi G, LaRocque JJ, Riedner B, Boly M, Postle BR, Tononi G, 2017. The neural correlates of dreaming . Nat. Neurosci 20 , 872–878. [ PMC free article ] [ PubMed ] [ Google Scholar ]
- Siclari F, LaRocque JJ, Postle BR, Tononi G, 2013. Assessing sleep consciousness within subjects using a serial awakening paradigm . Front. Psychol 4. [ PMC free article ] [ PubMed ] [ Google Scholar ]
- Snyder TJ, Gackenbach J, 1988. Individual differences associated with lucid dreaming, in: LaBerge S, Gackenbach J (Eds.), Conscious mind, sleeping brain . Plenum, New York, NY, pp. 221–259. [ Google Scholar ]
- Sparrow G, Hurd R, Carlson R, Molina A, 2018. Exploring the effects of galantamine paired with meditation and dream reliving on recalled dreams: Toward an integrated protocol for lucid dream induction and nightmare resolution . Conscious. Cogn 63 , 74–88. [ PubMed ] [ Google Scholar ]
- Sparrow GS, Thurston M, Carlson R, 2013. Dream reliving and meditation as a way to enhance reflectiveness and constructive engagement in dreams . Int. J. Dream Res 6 , 84–93. [ Google Scholar ]
- Spering M, Montagnini A, 2011. Do we track what we see? Common versus independent processing for motion perception and smooth pursuit eye movements: A review . Vision Res . 51 , 836–852. [ PubMed ] [ Google Scholar ]
- Spoormaker VI, Czisch M, Dresler M, 2010. Lucid and non-lucid dreaming: Thinking in networks . Int. J. Dream Res 3 , 49–51. [ Google Scholar ]
- Spoormaker VI, Van Den Bout J, 2006. Lucid dreaming treatment for nightmares: A pilot study . Psychother. Psychosom 75 , 389–394. [ PubMed ] [ Google Scholar ]
- Steriade M, Timofeev I, Grenier F, 2001. Natural waking and sleep states: A view from inside neocortical neurons . J. Neurophysiol 85 , 1969–1985. [ PubMed ] [ Google Scholar ]
- Stickgold R, Malia A, Fosse R, Hobson JA, 2001. Brain-mind states: I. Longitudinal field study of sleep/wake factors influencing mentation report length . Sleep 24 , 171–179. [ PubMed ] [ Google Scholar ]
- Stumbrys T, Erlacher D, 2012. Lucid dreaming during NREM sleep: Two case reports . Int. J. Dream Res 5 , 151–155. [ Google Scholar ]
- Stumbrys T, Erlacher D, Malinowski P, 2015. Meta-awareness during day and night: The relationship between mindfulness and lucid dreaming . Imagin. Cogn. Pers 34 , 415–433. [ Google Scholar ]
- Stumbrys T, Erlacher D, Schadlich M, Schredl M, 2012. Induction of lucid dreams: A systematic review of evidence . Conscious. Cogn 21 , 1456–1475. [ PubMed ] [ Google Scholar ]
- Stumbrys T, Erlacher D, Schredl M, 2013a. Reliability and stability of lucid dream and nightmare frequency scales . Int. J. Dream Res 6 , 123–126. [ Google Scholar ]
- Stumbrys T, Erlacher D, Schredl M, 2013b. Testing the involvement of the prefrontal cortex in lucid dreaming: A tDCS study . Conscious. Cogn 22 , 1214–1222. [ PubMed ] [ Google Scholar ]
- Tanner BA, 2004. Multimodal behavioral treatment of nonrepetitive, treatment-resistant nightmares: A case report . Percept. Motor Skills 99 , 1139–1146. [ PubMed ] [ Google Scholar ]
- Tononi G, 2011. Integrated information theory of consciousness: An updated account . Arch. Ital. Biol 150 , 56–90. [ PubMed ] [ Google Scholar ]
- Tononi G, Boly M, Massimini M, Koch C, 2016. Integrated information theory: From consciousness to its physical substrate . Nat. Rev. Neurosci 17 , 450–461. [ PubMed ] [ Google Scholar ]
- Tononi G, Massimini M, 2008. Why does consciousness fade in early sleep? Ann. N. Y. Acad. Sci 1129 , 330–334. [ PubMed ] [ Google Scholar ]
- Tyson PD, Ogilvie RD, Hunt HT, 1984. Lucid, prelucid, and nonlucid dreams related to the amount of EEG alpha activity during REM sleep . Psychophysiology 21 , 442–451. [ PubMed ] [ Google Scholar ]
- Van der Werf YD, Witter MP, Groenewegen HJ, 2002. The intralaminar and midline nuclei of the thalamus. Anatomical and functional evidence for participation in processes of arousal and awareness . Brain Res. Rev 39 , 107–140. [ PubMed ] [ Google Scholar ]
- Van Eeden F, 1913. A study of dreams , Proceedings of the Society for Psychical Research , pp. 431–461. [ Google Scholar ]
- van Gaal S, Ridderinkhof KR, Scholte HS, Lamme VA, 2010. Unconscious activation of the prefrontal no-go network . J. Neurosci 30 , 4143–4150. [ PMC free article ] [ PubMed ] [ Google Scholar ]
- Vanni S, Portin K, Virsu V, Hari R, 1999. Mu rhythm modulation during changes of visual percepts . Neuroscience 91 , 21–31. [ PubMed ] [ Google Scholar ]
- Velazquez-Moctezuma J, Shalauta M, Gillin JC, Shiromani PJ, 1991. Cholinergic antagonists and REM sleep generation . Brain Res . 543 , 175–179. [ PubMed ] [ Google Scholar ]
- Violante IR, Li LM, Carmichael DW, Lorenz R, Leech R, Hampshire A, Rothwell JC, Sharp DJ, 2017. Externally induced frontoparietal synchronization modulates network dynamics and enhances working memory performance . Elife 6 , e22001. [ PMC free article ] [ PubMed ] [ Google Scholar ]
- Vogel GW, 1975. A review of REM sleep deprivation . Arch. Gen. Psychiatry 32 , 749–761. [ PubMed ] [ Google Scholar ]
- Voss U, Holzmann R, Hobson A, Paulus W, Koppehele-Gossel J, Klimke A, Nitsche MA, 2014. Induction of self awareness in dreams through frontal low current stimulation of gamma activity . Nat. Neurosci 17 , 810–812. [ PubMed ] [ Google Scholar ]
- Voss U, Holzmann R, Tuin I, Hobson JA, 2009. Lucid dreaming: A state of consciousness with features of both waking and non-lucid dreaming . Sleep 32 , 1191–1200. [ PMC free article ] [ PubMed ] [ Google Scholar ]
- Voss U, Schermelleh-Engel K, Windt J, Frenzel C, Hobson A, 2013. Measuring consciousness in dreams: The lucidity and consciousness in dreams scale . Conscious. Cogn 22 , 8–21. [ PubMed ] [ Google Scholar ]
- Wagner AD, Shannon BJ, Kahn I, Buckner RL, 2005. Parietal lobe contributions to episodic memory retrieval . Trends Cog. Sci 9 , 445–453. [ PubMed ] [ Google Scholar ]
- Wallace BA, 2013. Buddhism and science: Breaking new ground . Columbia University Press. [ Google Scholar ]
- Wallace BA, Hodel B, 2012. Dreaming yourself awake: Lucid dreaming and Tibetan dream yoga for insight and transformation . Shambhala Publications, Boston, MA. [ Google Scholar ]
- Windt JM, 2010. The immersive spatiotemporal hallucination model of dreaming . Phenomenol. Cogn. Sci 9 , 295–316. [ Google Scholar ]
- Windt JM, 2015. Dreaming: A conceptual framework for philosophy of mind and empirical research . MIT Press, Cambridge, MA. [ Google Scholar ]
- Windt JM, Metzinger T, 2007. The philosophy of dreaming and self-consciousness: What happens to the experiential subject during the dream state?, in: Barrett D, McNamara P (Eds.), The new science of dreaming: Cultural and theoretical perspectives . Praeger; , Westport, CT, pp. 193–247. [ Google Scholar ]
- Windt JM, Nielsen T, Thompson E, 2016. Does consciousness disappear in dreamless sleep ? Trends Cog. Sci 20 , 871–882. [ PubMed ] [ Google Scholar ]
- Yeo B, Krienen FM, Sepulcre J, Sabuncu MR, Lashkari D, Hollinshead M, Roffman JL, Smoller JW, Zöllei L, Polimeni JR, 2011. The organization of the human cerebral cortex estimated by intrinsic functional connectivity . J. Neurophysiol 106 , 1125–1165. [ PMC free article ] [ PubMed ] [ Google Scholar ]
- Yuschak T, 2006. Advanced lucid dreaming: The power of supplements . Lulu Enterprises, Raleigh, NC. [ Google Scholar ]
- Yuval-Greenberg S, Deouell LY, 2009. The broadband-transient induced gamma-band response in scalp EEG reflects the execution of saccades . Brain Topogr . 22 , 3–6. [ PubMed ] [ Google Scholar ]
- Yuval-Greenberg S, Tomer O, Keren AS, Nelken I, Deouell LY, 2008. Transient induced gamma-band response in EEG as a manifestation of miniature saccades . Neuron 58 , 429–441. [ PubMed ] [ Google Scholar ]
- Zadra AL, Pihl RO, 1997. Lucid dreaming as a treatment for recurrent nightmares . Psychother. Psychosom 66 , 50–55. [ PubMed ] [ Google Scholar ]
- Bipolar Disorder
- Therapy Center
- When To See a Therapist
- Types of Therapy
- Best Online Therapy
- Best Couples Therapy
- Best Family Therapy
- Managing Stress
- Sleep and Dreaming
- Understanding Emotions
- Self-Improvement
- Healthy Relationships
- Student Resources
- Personality Types
- Guided Meditations
- Verywell Mind Insights
- 2024 Verywell Mind 25
- Mental Health in the Classroom
- Editorial Process
- Meet Our Review Board
- Crisis Support
Why Do We Dream?
There's no single consensus about which dream theory best explains why we dream
Kendra Cherry, MS, is a psychosocial rehabilitation specialist, psychology educator, and author of the "Everything Psychology Book."
:max_bytes(150000):strip_icc():format(webp)/IMG_9791-89504ab694d54b66bbd72cb84ffb860e.jpg)
Dr. Sabrina Romanoff, PsyD, is a licensed clinical psychologist and a professor at Yeshiva University’s clinical psychology doctoral program.
:max_bytes(150000):strip_icc():format(webp)/SabrinaRomanoffPhoto2-7320d6c6ffcc48ba87e1bad8cae3f79b.jpg)
Verywell / Madelyn Goodnight
What Is a Dream Theory?
- The Role of Dreams
- Reflect the Unconscious
- Process Information
- Aid In Memory
- Spur Creativity
- Reflect Your Life
- Prepare and Protect
- Process Emotions
- Other Theories
Lucid Dreaming
Stress dreams.
A dream theory is a proposed explanation for why people dream that is backed by scientific evidence. Despite scientific inquiry, we still don't have a solid answer for why people dream. Some of the most notable theories are that dreaming helps us process memories and better understand our emotions , also providing a way to express what we want or to practice facing our challenges.
At a Glance
There is no single dream theory that fully explains all of the aspects of why we dream. The most prominent theory is that dreams help us to process and consolidate information from the previous day. However, other theories have suggested that dreams are critical for emotional processing, creativity, and self-knowledge.
Some theories suggest that dreams also have symbolic meanings that offer a glimpse into the unconscious mind. Keep reading to learn more about some of the best-known theories about why we dream.
7 Theories on Why We Dream
A dream theory focuses on understanding the nature and purpose of dreams. Studying dreams can be challenging since they can vary greatly in how they are remembered and what they are about.
Dreams include the images, thoughts, and emotions that are experienced during sleep. They can range from extraordinarily intense or emotional to very vague, fleeting, confusing, or even boring.
Some dreams are joyful, while others are frightening or sad. Sometimes dreams seem to have a clear narrative, while many others appear to make no sense at all.
There are many unknowns about dreaming and sleep, but what scientists do know is that just about everyone dreams every time they sleep, for a total of around two hours per night, whether they remember it upon waking or not .
Beyond what's in a particular dream, there is the question of why we dream at all. Below, we detail the most prominent theories on the purpose of dreaming and how these explanations can be applied to specific dreams.
How Do Scientists Study Dreams?
The question of why we dream has fascinated philosophers and scientists for thousands of years. Traditionally, dream content is measured by the subjective recollections of the dreamer upon waking. However, observation is also accomplished through objective evaluation in a lab.
In one study, researchers even created a rudimentary dream content map that was able to track what people dreamed about in real time using magnetic resonance imaging (MRI) patterns. The map was then backed up by the dreamers' reports upon waking.
What Dream Theory Suggests About the Role of Dreams
Some of the more prominent dream theories suggest that the reason we dream is to:
- Consolidate memories
- Process emotions
- Express our deepest desires
- Gain practice confronting potential dangers
Many experts believe that we dream due to a combination of these reasons rather than any one particular theory. Additionally, while many researchers believe that dreaming is essential to mental, emotional, and physical well-being, some scientists suggest that dreams serve no real purpose at all.
The bottom line is that while many theories have been proposed, no single consensus has emerged about which dream theory best explains why we dream.
Dreaming during different phases of sleep may also serve unique purposes. The most vivid dreams happen during rapid eye movement (REM) sleep , and these are the dreams that we're most likely to recall. We also dream during non-rapid eye movement (non-REM) sleep, but those dreams are known to be remembered less often and have more mundane content.
Sigmund Freud's Dream Theory
Sigmund Freud’s theory of dreams suggests that dreams represent unconscious desires, thoughts, wish fulfillment, and motivations. According to Freud, people are driven by repressed and unconscious longings, such as aggressive and sexual instincts .
While many of Freud's assertions have been debunked, research suggests there is a dream rebound effect, also known as dream rebound theory, in which suppression of a thought tends to result in dreaming about it.
What Causes Dreams to Happen?
In " The Interpretation of Dreams ," Freud wrote that dreams are "disguised fulfillments of repressed wishes." He also described two different components of dreams: manifest content (actual images) and latent content (hidden meaning).
Freud’s theory contributed to the rise and popularity of dream interpretation . While research has failed to demonstrate that the manifest content disguises the psychological significance of a dream, some experts believe that dreams play an important role in processing emotions and stressful experiences.
Activation-Synthesis Dream Theory
According to the activation-synthesis model of dreaming , which was first proposed by J. Allan Hobson and Robert McCarley, circuits in the brain become activated during REM sleep, which triggers the amygdala and hippocampus to create an array of electrical impulses. This results in a compilation of random thoughts, images, and memories that appear while dreaming.
When we wake, our active minds pull together the dream's various images and memory fragments to create a cohesive narrative.
In the activation-synthesis hypothesis, dreams are a compilation of randomness that appear to the sleeping mind and are brought together in a meaningful way when we wake. In this sense, dreams may provoke the dreamer to make new connections, inspire useful ideas, or have creative epiphanies in their waking lives.
Self-Organization Dream Theory
According to the information-processing theory, sleep allows us to consolidate and process all of the information and memories that we have collected during the previous day. Some dream experts suggest that dreaming is a byproduct, or even an active part, of this experience processing.
This model, known as the self-organization theory of dreaming , explains that dreaming is a side effect of brain neural activity as memories are consolidated during sleep.
During this process of unconscious information redistribution, it is suggested that memories are either strengthened or weakened. According to the self-organization theory of dreaming, while we dream, helpful memories are made stronger, while less useful ones fade away.
Research supports this theory, finding improvement in complex tasks when a person dreams about doing them. Studies also show that during REM sleep, low-frequency theta waves were more active in the frontal lobe, just like they are when people are learning, storing, and remembering information when awake.
Creativity and Problem-Solving Dream Theory
Another theory about dreams says that their purpose is to help us solve problems. In this creativity theory of dreaming, the unconstrained, unconscious mind is free to wander its limitless potential while unburdened by the often stifling realities of the conscious world. In fact, research has shown dreaming to be an effective promoter of creative thinking.
Scientific research and anecdotal evidence back up the fact that many people do successfully mine their dreams for inspiration and credit their dreams for their big "aha" moments.
The ability to make unexpected connections between memories and ideas that appear in your dreams often proves to be an especially fertile ground for creativity.
Continuity Hypothesis Dream Theory
Under the continuity hypothesis, dreams function as a reflection of a person's real life, incorporating conscious experiences into their dreams. Rather than a straightforward replay of waking life, dreams show up as a patchwork of memory fragments.
Still, studies show that non-REM sleep may be more involved with declarative memory (the more routine stuff), while REM dreams include more emotional and instructive memories.
In general, REM dreams tend to be easier to recall compared to non-REM dreams.
Under the continuity hypothesis, memories may be fragmented purposefully in our dreams as part of incorporating new learning and experiences into long-term memory . Still, there are many unanswered questions as to why some aspects of memories are featured more or less prominently in our dreams.
Rehearsal and Adaptation Dream Theory
The primitive instinct rehearsal and adaptive strategy theories of dreaming propose that we dream to better prepare ourselves to confront dangers in the real world. The dream as a social simulation function or threat simulation provides the dreamer a safe environment to practice important survival skills.
While dreaming, we hone our fight-or-flight instincts and build mental capability for handling threatening scenarios. Under the threat simulation theory, our sleeping brains focus on the fight-or-flight mechanism to prep us for life-threatening and/or emotionally intense scenarios including:
- Running away from a pursuer
- Falling over a cliff
- Showing up somewhere naked
- Going to the bathroom in public
- Forgetting to study for a final exam
This theory suggests that practicing or rehearsing these skills in our dreams gives us an evolutionary advantage in that we can better cope with or avoid threatening scenarios in the real world. This helps explain why so many dreams contain scary, dramatic, or intense content.
Emotional Regulation Dream Theory
The emotional regulation dream theory says that the function of dreams is to help us process and cope with our emotions or trauma in the safe space of slumber.
Research shows that the amygdala , which is involved in processing emotions, and the hippocampus , which plays a vital role in condensing information and moving it from short-term to long-term memory storage, are active during vivid, intense dreaming.
This illustrates a strong link between dreaming, memory storage, and emotional processing.
This theory suggests that REM sleep plays a vital role in emotional brain regulation. It also helps explain why so many dreams are emotionally vivid and why emotional or traumatic experiences tend to show up on repeat. Research has shown a connection between the ability to process emotions and the amount of REM sleep a person gets.
Sharing Dreams Promotes Connection
Talking about content similarities and common dreams with others may help promote belongingness and connection. Research notes heightened empathy among people who share their dreams with others, pointing to another way dreams can help us cope by promoting community and interpersonal support.
Other Theories About Why We Dream
Many other theories have been suggested to account for why we dream.
- One dream theory contends that dreams are the result of our brains trying to interpret external stimuli (such as a dog's bark, music, or a baby's cry) during sleep.
- Another theory uses a computer metaphor to account for dreams, noting that dreams serve to "clean up" clutter from the mind, refreshing the brain for the next day.
- The reverse-learning theory suggests that we dream to forget. Our brains have thousands of neural connections between memories—too many to remember them all—and that dreaming is part of "pruning" those connections.
- In the continual-activation theory, we dream to keep the brain active while we sleep, in order to keep it functioning properly.
Overfitted Dream Hypothesis
One recently introduced dream theory, known as the overfitted dream hypothesis, suggests that dreams are the brain's way of introducing random, disruptive data to help break up repetitive daily tasks and information. Researcher Erik Hoel suggests that such disruptions helps to keep the brain fit.
Lucid dreams are relatively rare dreams where the dreamer has awareness of being in their dream and often has some control over the dream content. Research indicates that around 50% of people recall having had at least one lucid dream in their lifetime and just over 10% report having them two or more times per month.
It is unknown why certain people experience lucid dreams more frequently than others. While experts are unclear as to why or how lucid dreaming occurs, preliminary research signals that the prefrontal and parietal regions of the brain play a significant role.
How to Lucid Dream
Many people covet lucid dreaming and seek to experience it more often. Lucid dreaming has been compared to virtual reality and hyper-realistic video games, giving lucid dreamers the ultimate self-directed dreamscape experience.
Potential training methods for inducing lucid dreaming include cognitive training, external stimulation during sleep, and medications. While these methods may show some promise, none have been rigorously tested or shown to be effective.
A strong link has been found between lucid dreaming and highly imaginative thinking and creative output. Research has shown that lucid dreamers perform better on creative tasks than those who do not experience lucid dreaming.
Stressful experiences tend to show up with great frequency in our dreams. Stress dreams may be described as sad, scary, and nightmarish .
Experts do not fully understand how or why specific stressful content ends up in our dreams, but many point to a variety of theories, including the continuity hypothesis, adaptive strategy, and emotional regulation dream theories to explain these occurrences. Stress dreams and mental health seem to go hand-in-hand.
- Daily stress shows up in dreams : Research has shown that those who experience greater levels of worry in their waking lives and people diagnosed with post-traumatic stress disorder (PTSD) report higher frequency and intensity of nightmares.
- Mental health disorders may contribute to stress dreams : Those with mental health disorders such as anxiety, bipolar disorder , and depression tend to have more distressing dreams, as well as more difficulty sleeping in general.
- Anxiety is linked to stress dreams : Research indicates a strong connection between anxiety and stressful dream content. These dreams may be the brain's attempt to help us cope with and make sense of these stressful experiences.
While many theories exist about why we dream, more research is needed to fully understand their purpose. Rather than assuming only one dream theory is correct, dreams likely serve various purposes. In reality, many of these dream theories may be useful for explaining different aspects of the dreaming process.
If you are concerned about your dreams and/or are having frequent nightmares , consider speaking to your doctor or consulting a sleep specialist.
National Institute of Neurological Disorders and Stroke. Brain basics: Understanding sleep .
Horikawa T, Tamaki M, Miyawaki Y, Kamitani Y. Neural decoding of visual imagery during sleep . Science . 2013;340(6132):639-42. doi:10.1126/science.1234330
De Gennaro L, Cipolli C, Cherubini A, et al. Amygdala and hippocampus volumetry and diffusivity in relation to dreaming . Hum Brain Mapp . 2011;32(9):1458-70. doi:10.1002/hbm.21120
Zhang W, Guo B. Freud's dream interpretation: A different perspective based on the self-organization theory of dreaming . Front Psychol . 2018;9:1553. doi:10.3389/fpsyg.2018.01553
Malinowski J, Carr M, Edwards C, Ingarfill A, Pinto A. The effects of dream rebound: evidence for emotion-processing theories of dreaming . J Sleep Res . 2019;28(5):e12827. doi:10.1111/jsr.12827
Boag S. On dreams and motivation: Comparison of Freud's and Hobson's views . Front Psychol . 2017;7:2001. doi:10.3389/fpsyg.2016.02001
Eichenlaub JB, Van Rijn E, Gaskell MG, et al. Incorporation of recent waking-life experiences in dreams correlates with frontal theta activity in REM sleep . Soc Cogn Affect Neurosci . 2018;13(6):637-647. doi:10.1093/scan/nsy041
Zhang W. A supplement to self-organization theory of dreaming . Front Psychol . 2016;7. doi:10.3389/fpsyg.2016.00332
Luongo A, Lukowski A, Protho T, Van Vorce H, Pisani L, Edgin J. Sleep's role in memory consolidation: What can we learn from atypical development ? Adv Child Dev Behav . 2021;60:229-260. doi:10.1016/bs.acdb.2020.08.001
Scarpelli S, D'Atri A, Gorgoni M, Ferrara M, De Gennaro L. EEG oscillations during sleep and dream recall: state- or trait-like individual differences ? Front Psychol . 2015;6:605. doi:10.3389/fpsyg.2015.00605
Llewellyn S, Desseilles M. Editorial: Do both psychopathology and creativity result from a labile wake-sleep-dream cycle? . Front Psychol . 2017;8:1824. doi:10.3389/fpsyg.2017.01824
Revonsuo A. The reinterpretation of dreams: An evolutionary hypothesis of the function of dreaming . Behav Brain Sci . 2000;23(6):877-901. doi:10.1017/s0140525x00004015
Ruby PM. Experimental research on dreaming: State of the art and neuropsychoanalytic perspectives . Front Psychol . 2011;2:286. doi:10.3389/fpsyg.2011.00286
Gujar N, McDonald SA, Nishida M, Walker MP. A role for REM sleep in recalibrating the sensitivity of the human brain to specific emotions . Cereb Cortex . 2011;21(1):115-23. doi:10.1093/cercor/bhq064
Blagrove M, Hale S, Lockheart J, Carr M, Jones A, Valli K. Testing the empathy theory of dreaming: The relationships between dream sharing and trait and state empathy . Front Psychol . 2019;10:1351. doi:10.3389/fpsyg.2019.01351
Poe GR. Sleep is for forgetting . J Neurosci . 2017;37(3):464-473. doi:10.1523/JNEUROSCI.0820-16.2017
Zhang J. Continual-activation theory of dreaming . Dynamical Psychology .
Hoel E. The Overfitted Brain: Dreams evolved to assist generalization . Published online 2020.doi:10.48550/arXiv.2007.09560
Vallat R, Ruby PM. Is it a good idea to cultivate lucid dreaming? Front Psychol . 2019;10:2585. doi:10.3389/fpsyg.2019.02585
Baird B, Mota-Rolim SA, Dresler M. The cognitive neuroscience of lucid dreaming . Neurosci Biobehav Rev . 2019;100:305-323. doi:10.1016/j.neubiorev.2019.03.008
Stumbrys T, Daunytė V. Visiting the land of dream muses: The relationship between lucid dreaming and creativity . 2018;11(2). doi:10.11588/ijodr.2018.2.48667
Rek S, Sheaves B, Freeman D. Nightmares in the general population: Identifying potential causal factors . Soc Psychiatry Psychiatr Epidemiol . 2017;52(9):1123-1133. doi:10.1007/s00127-017-1408-7
Sikka P, Pesonen H, Revonsuo A. Peace of mind and anxiety in the waking state are related to the affective content of dreams . Sci Rep . 2018;8(1):12762. doi:10.1038/s41598-018-30721-1
By Kendra Cherry, MSEd Kendra Cherry, MS, is a psychosocial rehabilitation specialist, psychology educator, and author of the "Everything Psychology Book."
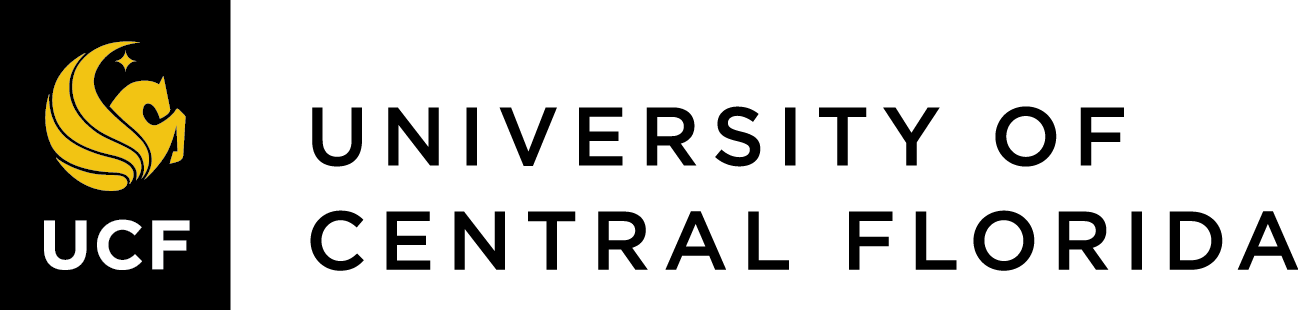
States of Consciousness
Dreams and Dreaming
Learning objectives.
- Describe and differentiate between theories on why we dream
The meaning of dreams varies across different cultures and periods of time. By the late 19th century, German psychiatrist Sigmund Freud had become convinced that dreams represented an opportunity to gain access to the unconscious. By analyzing dreams, Freud thought people could increase self-awareness and gain valuable insight to help them deal with the problems they faced in their lives. Freud made distinctions between the manifest content and the latent content of dreams.
Manifest content is the actual content, or storyline, of a dream. Latent content , on the other hand, refers to the hidden meaning of a dream. For instance, if a woman dreams about being chased by a snake, Freud might have argued that this represents the woman’s fear of sexual intimacy, with the snake serving as a symbol of a man’s penis.
Freud was not the only theorist to focus on the content of dreams. The 20th century Swiss psychiatrist Carl Jung believed that dreams allowed us to tap into the collective unconscious . The collective unconscious, as described by Jung, is a theoretical repository of information he believed to be shared by everyone. According to Jung, certain symbols in dreams reflected universal archetypes with meanings that are similar for all people regardless of culture or location.
The sleep and dreaming researcher Rosalind Cartwright, however, believes that dreams simply reflect life events that are important to the dreamer. Unlike Freud and Jung, Cartwright’s ideas about dreaming have found empirical support. For example, she and her colleagues published a study in which women going through divorce were asked several times over a five month period to report the degree to which their former spouses were on their minds. These same women were awakened during REM sleep in order to provide a detailed account of their dream content. There was a significant positive correlation between the degree to which women thought about their former spouses during waking hours and the number of times their former spouses appeared as characters in their dreams (Cartwright, Agargun, Kirkby, & Friedman, 2006). Recent research (Horikawa, Tamaki, Miyawaki, & Kamitani, 2013) has uncovered new techniques by which researchers may effectively detect and classify the visual images that occur during dreaming by using fMRI for neural measurement of brain activity patterns, opening the way for additional research in this area.
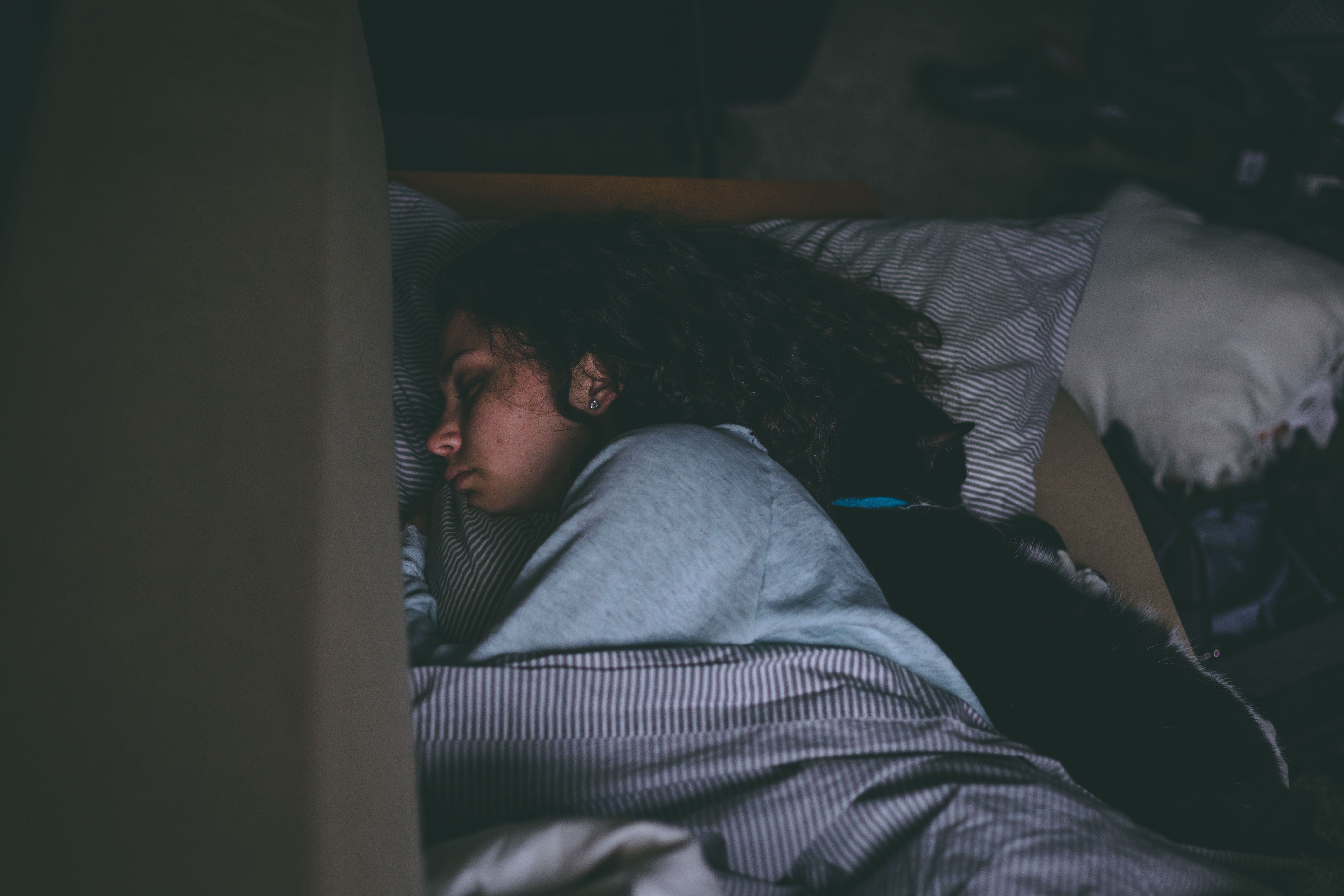
Recently, neuroscientists have also become interested in understanding why we dream. For example, Hobson (2009) suggests that dreaming may represent a state of protoconsciousness. In other words, dreaming involves constructing a virtual reality in our heads that we might use to help us during wakefulness. Among a variety of neurobiological evidence, John Hobson cites research on lucid dreams as an opportunity to better understand dreaming in general. Lucid dreams are dreams in which certain aspects of wakefulness are maintained during a dream state. In a lucid dream, a person becomes aware of the fact that they are dreaming, and as such, they can control the dream’s content (LaBerge, 1990).
Theories on Dreaming
While the Freudian theory of dreaming may be the most well known, and Cartwright’s suggestions on dreaming the most plausible, there are several other theories about the purpose of dreaming. The threat-simulation theory suggests that dreaming should be seen as an ancient biological defense mechanism. Dreams are thought to provide an evolutionary advantage because of their capacity to repeatedly simulate potential threatening events. This process enhances the neurocognitive mechanisms required for efficient threat perception and avoidance.
The expectation-fulfillment theory posits that dreaming serves to discharge emotional arousals (however minor) that haven’t been expressed during the day. This practice frees up space in the brain to deal with the emotional arousals of the next day and allows instinctive urges to stay intact. In effect, the expectation is fulfilled (the action is “completed”) in a metaphorical form so that a false memory is not created. This theory explains why dreams are usually forgotten immediately afterwards.
One prominent neurobiological theory of dreaming is the activation-synthesis theory , which states that dreams don’t actually mean anything. They are merely electrical brain impulses that pull random thoughts and imagery from our memories. The theory posits that humans construct dream stories after they wake up, in a natural attempt to make sense of the nonsensical. However, given the vast documentation of the realistic aspects of human dreaming, as well as indirect experimental evidence that other mammals such as cats also dream, evolutionary psychologists have theorized that dreaming does indeed serve a purpose.
The continual-activation theory proposes that dreaming is a result of brain activation and synthesis. Dreaming and REM sleep are simultaneously controlled by different brain mechanisms. The hypothesis states that the function of sleep is to process, encode, and transfer data from short-term memory to long-term memory through a process called consolidation. However, there is not much evidence to back this up. NREM sleep processes the conscious-related memory (declarative memory), and REM sleep processes the unconscious related memory (procedural memory).
The underlying assumption of continual-activation theory is that, during REM sleep, the unconscious part of the brain is busy processing procedural memory. Meanwhile, the level of activation in the conscious part of the brain descends to a very low level as the inputs from the senses are basically disconnected. This triggers the “continual-activation” mechanism to generate a data stream from the memory stores to flow through to the conscious part of the brain.
Link to Learning
Review the purpose and stages of sleep as well as the reasons why we dream in the following CrashCourse video:
You can view the transcript for “To Sleep, Perchance to Dream: Crash Course Psychology #9” here (opens in new window) .
CC licensed content, Original
- Modification, adaptation, and original content. Provided by : Lumen Learning. License : CC BY: Attribution
CC licensed content, Shared previously
- Stages of Sleep. Authored by : OpenStax College. Located at : https://openstax.org/books/psychology-2e/pages/4-3-stages-of-sleep . License : CC BY: Attribution . License Terms : Download for free at https://openstax.org/books/psychology-2e/pages/1-introduction
- The Nature and Meaning of Dreams. Provided by : Boundless. Located at : https://www.boundless.com/psychology/textbooks/boundless-psychology-textbook/states-of-consciousness-6/sleep-and-dreaming-42/the-nature-and-meaning-of-dreams-184-12719/ . License : CC BY-SA: Attribution-ShareAlike
- Sleeping woman. Authored by : Craig Adderley. Provided by : Pexels. Located at : https://www.pexels.com/photo/woman-sleeping-1497855/ . License : CC0: No Rights Reserved
All rights reserved content
- To Sleep, Perchance to Dream – Crash Course Psychology #9. Provided by : CrashCourse. Located at : https://www.youtube.com/watch?v=rMHus-0wFSo . License : Other . License Terms : Standard YouTube License
storyline of events that occur during a dream, per Sigmund Freud’s view of the function of dreams
hidden meaning of a dream, per Sigmund Freud’s view of the function of dreams
common psychological tendencies that have been passed down from one generation to the next
people become aware that they are dreaming and can control the dream’s content
suggests that dreaming should be seen as an ancient biological defense mechanism that provides an evolutionary advantage because of its capacity to repeatedly simulate potential threatening events, thus enhancing the mechanisms required for efficient threat avoidance.
states that dreams don't actually mean anything. Instead, dreams are merely electrical brain impulses that pull random thoughts and imagery from our memories.
proposes that dreaming is a result of brain activation and synthesis; its assumption is that, during REM sleep, the unconscious part of the brain is busy processing procedural memory
General Psychology Copyright © by OpenStax and Lumen Learning is licensed under a Creative Commons Attribution 4.0 International License , except where otherwise noted.
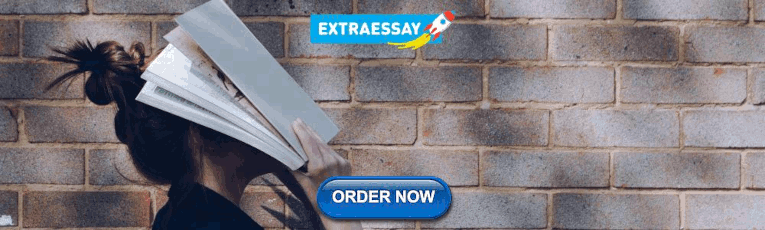
Share This Book
- Search Menu
- Sign in through your institution
- Browse content in Arts and Humanities
- Browse content in Archaeology
- Anglo-Saxon and Medieval Archaeology
- Archaeological Methodology and Techniques
- Archaeology by Region
- Archaeology of Religion
- Archaeology of Trade and Exchange
- Biblical Archaeology
- Contemporary and Public Archaeology
- Environmental Archaeology
- Historical Archaeology
- History and Theory of Archaeology
- Industrial Archaeology
- Landscape Archaeology
- Mortuary Archaeology
- Prehistoric Archaeology
- Underwater Archaeology
- Zooarchaeology
- Browse content in Architecture
- Architectural Structure and Design
- History of Architecture
- Residential and Domestic Buildings
- Theory of Architecture
- Browse content in Art
- Art Subjects and Themes
- History of Art
- Industrial and Commercial Art
- Theory of Art
- Biographical Studies
- Byzantine Studies
- Browse content in Classical Studies
- Classical History
- Classical Philosophy
- Classical Mythology
- Classical Literature
- Classical Reception
- Classical Art and Architecture
- Classical Oratory and Rhetoric
- Greek and Roman Epigraphy
- Greek and Roman Law
- Greek and Roman Papyrology
- Greek and Roman Archaeology
- Late Antiquity
- Religion in the Ancient World
- Digital Humanities
- Browse content in History
- Colonialism and Imperialism
- Diplomatic History
- Environmental History
- Genealogy, Heraldry, Names, and Honours
- Genocide and Ethnic Cleansing
- Historical Geography
- History by Period
- History of Emotions
- History of Agriculture
- History of Education
- History of Gender and Sexuality
- Industrial History
- Intellectual History
- International History
- Labour History
- Legal and Constitutional History
- Local and Family History
- Maritime History
- Military History
- National Liberation and Post-Colonialism
- Oral History
- Political History
- Public History
- Regional and National History
- Revolutions and Rebellions
- Slavery and Abolition of Slavery
- Social and Cultural History
- Theory, Methods, and Historiography
- Urban History
- World History
- Browse content in Language Teaching and Learning
- Language Learning (Specific Skills)
- Language Teaching Theory and Methods
- Browse content in Linguistics
- Applied Linguistics
- Cognitive Linguistics
- Computational Linguistics
- Forensic Linguistics
- Grammar, Syntax and Morphology
- Historical and Diachronic Linguistics
- History of English
- Language Acquisition
- Language Evolution
- Language Reference
- Language Variation
- Language Families
- Lexicography
- Linguistic Anthropology
- Linguistic Theories
- Linguistic Typology
- Phonetics and Phonology
- Psycholinguistics
- Sociolinguistics
- Translation and Interpretation
- Writing Systems
- Browse content in Literature
- Bibliography
- Children's Literature Studies
- Literary Studies (Asian)
- Literary Studies (European)
- Literary Studies (Eco-criticism)
- Literary Studies (Romanticism)
- Literary Studies (American)
- Literary Studies (Modernism)
- Literary Studies - World
- Literary Studies (1500 to 1800)
- Literary Studies (19th Century)
- Literary Studies (20th Century onwards)
- Literary Studies (African American Literature)
- Literary Studies (British and Irish)
- Literary Studies (Early and Medieval)
- Literary Studies (Fiction, Novelists, and Prose Writers)
- Literary Studies (Gender Studies)
- Literary Studies (Graphic Novels)
- Literary Studies (History of the Book)
- Literary Studies (Plays and Playwrights)
- Literary Studies (Poetry and Poets)
- Literary Studies (Postcolonial Literature)
- Literary Studies (Queer Studies)
- Literary Studies (Science Fiction)
- Literary Studies (Travel Literature)
- Literary Studies (War Literature)
- Literary Studies (Women's Writing)
- Literary Theory and Cultural Studies
- Mythology and Folklore
- Shakespeare Studies and Criticism
- Browse content in Media Studies
- Browse content in Music
- Applied Music
- Dance and Music
- Ethics in Music
- Ethnomusicology
- Gender and Sexuality in Music
- Medicine and Music
- Music Cultures
- Music and Religion
- Music and Media
- Music and Culture
- Music Education and Pedagogy
- Music Theory and Analysis
- Musical Scores, Lyrics, and Libretti
- Musical Structures, Styles, and Techniques
- Musicology and Music History
- Performance Practice and Studies
- Race and Ethnicity in Music
- Sound Studies
- Browse content in Performing Arts
- Browse content in Philosophy
- Aesthetics and Philosophy of Art
- Epistemology
- Feminist Philosophy
- History of Western Philosophy
- Metaphysics
- Moral Philosophy
- Non-Western Philosophy
- Philosophy of Science
- Philosophy of Language
- Philosophy of Mind
- Philosophy of Perception
- Philosophy of Action
- Philosophy of Law
- Philosophy of Religion
- Philosophy of Mathematics and Logic
- Practical Ethics
- Social and Political Philosophy
- Browse content in Religion
- Biblical Studies
- Christianity
- East Asian Religions
- History of Religion
- Judaism and Jewish Studies
- Qumran Studies
- Religion and Education
- Religion and Health
- Religion and Politics
- Religion and Science
- Religion and Law
- Religion and Art, Literature, and Music
- Religious Studies
- Browse content in Society and Culture
- Cookery, Food, and Drink
- Cultural Studies
- Customs and Traditions
- Ethical Issues and Debates
- Hobbies, Games, Arts and Crafts
- Natural world, Country Life, and Pets
- Popular Beliefs and Controversial Knowledge
- Sports and Outdoor Recreation
- Technology and Society
- Travel and Holiday
- Visual Culture
- Browse content in Law
- Arbitration
- Browse content in Company and Commercial Law
- Commercial Law
- Company Law
- Browse content in Comparative Law
- Systems of Law
- Competition Law
- Browse content in Constitutional and Administrative Law
- Government Powers
- Judicial Review
- Local Government Law
- Military and Defence Law
- Parliamentary and Legislative Practice
- Construction Law
- Contract Law
- Browse content in Criminal Law
- Criminal Procedure
- Criminal Evidence Law
- Sentencing and Punishment
- Employment and Labour Law
- Environment and Energy Law
- Browse content in Financial Law
- Banking Law
- Insolvency Law
- History of Law
- Human Rights and Immigration
- Intellectual Property Law
- Browse content in International Law
- Private International Law and Conflict of Laws
- Public International Law
- IT and Communications Law
- Jurisprudence and Philosophy of Law
- Law and Politics
- Law and Society
- Browse content in Legal System and Practice
- Courts and Procedure
- Legal Skills and Practice
- Primary Sources of Law
- Regulation of Legal Profession
- Medical and Healthcare Law
- Browse content in Policing
- Criminal Investigation and Detection
- Police and Security Services
- Police Procedure and Law
- Police Regional Planning
- Browse content in Property Law
- Personal Property Law
- Study and Revision
- Terrorism and National Security Law
- Browse content in Trusts Law
- Wills and Probate or Succession
- Browse content in Medicine and Health
- Browse content in Allied Health Professions
- Arts Therapies
- Clinical Science
- Dietetics and Nutrition
- Occupational Therapy
- Operating Department Practice
- Physiotherapy
- Radiography
- Speech and Language Therapy
- Browse content in Anaesthetics
- General Anaesthesia
- Neuroanaesthesia
- Browse content in Clinical Medicine
- Acute Medicine
- Cardiovascular Medicine
- Clinical Genetics
- Clinical Pharmacology and Therapeutics
- Dermatology
- Endocrinology and Diabetes
- Gastroenterology
- Genito-urinary Medicine
- Geriatric Medicine
- Infectious Diseases
- Medical Toxicology
- Medical Oncology
- Pain Medicine
- Palliative Medicine
- Rehabilitation Medicine
- Respiratory Medicine and Pulmonology
- Rheumatology
- Sleep Medicine
- Sports and Exercise Medicine
- Clinical Neuroscience
- Community Medical Services
- Critical Care
- Emergency Medicine
- Forensic Medicine
- Haematology
- History of Medicine
- Browse content in Medical Dentistry
- Oral and Maxillofacial Surgery
- Paediatric Dentistry
- Restorative Dentistry and Orthodontics
- Surgical Dentistry
- Browse content in Medical Skills
- Clinical Skills
- Communication Skills
- Nursing Skills
- Surgical Skills
- Medical Ethics
- Medical Statistics and Methodology
- Browse content in Neurology
- Clinical Neurophysiology
- Neuropathology
- Nursing Studies
- Browse content in Obstetrics and Gynaecology
- Gynaecology
- Occupational Medicine
- Ophthalmology
- Otolaryngology (ENT)
- Browse content in Paediatrics
- Neonatology
- Browse content in Pathology
- Chemical Pathology
- Clinical Cytogenetics and Molecular Genetics
- Histopathology
- Medical Microbiology and Virology
- Patient Education and Information
- Browse content in Pharmacology
- Psychopharmacology
- Browse content in Popular Health
- Caring for Others
- Complementary and Alternative Medicine
- Self-help and Personal Development
- Browse content in Preclinical Medicine
- Cell Biology
- Molecular Biology and Genetics
- Reproduction, Growth and Development
- Primary Care
- Professional Development in Medicine
- Browse content in Psychiatry
- Addiction Medicine
- Child and Adolescent Psychiatry
- Forensic Psychiatry
- Learning Disabilities
- Old Age Psychiatry
- Psychotherapy
- Browse content in Public Health and Epidemiology
- Epidemiology
- Public Health
- Browse content in Radiology
- Clinical Radiology
- Interventional Radiology
- Nuclear Medicine
- Radiation Oncology
- Reproductive Medicine
- Browse content in Surgery
- Cardiothoracic Surgery
- Gastro-intestinal and Colorectal Surgery
- General Surgery
- Neurosurgery
- Paediatric Surgery
- Peri-operative Care
- Plastic and Reconstructive Surgery
- Surgical Oncology
- Transplant Surgery
- Trauma and Orthopaedic Surgery
- Vascular Surgery
- Browse content in Science and Mathematics
- Browse content in Biological Sciences
- Aquatic Biology
- Biochemistry
- Bioinformatics and Computational Biology
- Developmental Biology
- Ecology and Conservation
- Evolutionary Biology
- Genetics and Genomics
- Microbiology
- Molecular and Cell Biology
- Natural History
- Plant Sciences and Forestry
- Research Methods in Life Sciences
- Structural Biology
- Systems Biology
- Zoology and Animal Sciences
- Browse content in Chemistry
- Analytical Chemistry
- Computational Chemistry
- Crystallography
- Environmental Chemistry
- Industrial Chemistry
- Inorganic Chemistry
- Materials Chemistry
- Medicinal Chemistry
- Mineralogy and Gems
- Organic Chemistry
- Physical Chemistry
- Polymer Chemistry
- Study and Communication Skills in Chemistry
- Theoretical Chemistry
- Browse content in Computer Science
- Artificial Intelligence
- Computer Architecture and Logic Design
- Game Studies
- Human-Computer Interaction
- Mathematical Theory of Computation
- Programming Languages
- Software Engineering
- Systems Analysis and Design
- Virtual Reality
- Browse content in Computing
- Business Applications
- Computer Security
- Computer Games
- Computer Networking and Communications
- Digital Lifestyle
- Graphical and Digital Media Applications
- Operating Systems
- Browse content in Earth Sciences and Geography
- Atmospheric Sciences
- Environmental Geography
- Geology and the Lithosphere
- Maps and Map-making
- Meteorology and Climatology
- Oceanography and Hydrology
- Palaeontology
- Physical Geography and Topography
- Regional Geography
- Soil Science
- Urban Geography
- Browse content in Engineering and Technology
- Agriculture and Farming
- Biological Engineering
- Civil Engineering, Surveying, and Building
- Electronics and Communications Engineering
- Energy Technology
- Engineering (General)
- Environmental Science, Engineering, and Technology
- History of Engineering and Technology
- Mechanical Engineering and Materials
- Technology of Industrial Chemistry
- Transport Technology and Trades
- Browse content in Environmental Science
- Applied Ecology (Environmental Science)
- Conservation of the Environment (Environmental Science)
- Environmental Sustainability
- Environmentalist Thought and Ideology (Environmental Science)
- Management of Land and Natural Resources (Environmental Science)
- Natural Disasters (Environmental Science)
- Nuclear Issues (Environmental Science)
- Pollution and Threats to the Environment (Environmental Science)
- Social Impact of Environmental Issues (Environmental Science)
- History of Science and Technology
- Browse content in Materials Science
- Ceramics and Glasses
- Composite Materials
- Metals, Alloying, and Corrosion
- Nanotechnology
- Browse content in Mathematics
- Applied Mathematics
- Biomathematics and Statistics
- History of Mathematics
- Mathematical Education
- Mathematical Finance
- Mathematical Analysis
- Numerical and Computational Mathematics
- Probability and Statistics
- Pure Mathematics
- Browse content in Neuroscience
- Cognition and Behavioural Neuroscience
- Development of the Nervous System
- Disorders of the Nervous System
- History of Neuroscience
- Invertebrate Neurobiology
- Molecular and Cellular Systems
- Neuroendocrinology and Autonomic Nervous System
- Neuroscientific Techniques
- Sensory and Motor Systems
- Browse content in Physics
- Astronomy and Astrophysics
- Atomic, Molecular, and Optical Physics
- Biological and Medical Physics
- Classical Mechanics
- Computational Physics
- Condensed Matter Physics
- Electromagnetism, Optics, and Acoustics
- History of Physics
- Mathematical and Statistical Physics
- Measurement Science
- Nuclear Physics
- Particles and Fields
- Plasma Physics
- Quantum Physics
- Relativity and Gravitation
- Semiconductor and Mesoscopic Physics
- Browse content in Psychology
- Affective Sciences
- Clinical Psychology
- Cognitive Psychology
- Cognitive Neuroscience
- Criminal and Forensic Psychology
- Developmental Psychology
- Educational Psychology
- Evolutionary Psychology
- Health Psychology
- History and Systems in Psychology
- Music Psychology
- Neuropsychology
- Organizational Psychology
- Psychological Assessment and Testing
- Psychology of Human-Technology Interaction
- Psychology Professional Development and Training
- Research Methods in Psychology
- Social Psychology
- Browse content in Social Sciences
- Browse content in Anthropology
- Anthropology of Religion
- Human Evolution
- Medical Anthropology
- Physical Anthropology
- Regional Anthropology
- Social and Cultural Anthropology
- Theory and Practice of Anthropology
- Browse content in Business and Management
- Business Strategy
- Business Ethics
- Business History
- Business and Government
- Business and Technology
- Business and the Environment
- Comparative Management
- Corporate Governance
- Corporate Social Responsibility
- Entrepreneurship
- Health Management
- Human Resource Management
- Industrial and Employment Relations
- Industry Studies
- Information and Communication Technologies
- International Business
- Knowledge Management
- Management and Management Techniques
- Operations Management
- Organizational Theory and Behaviour
- Pensions and Pension Management
- Public and Nonprofit Management
- Strategic Management
- Supply Chain Management
- Browse content in Criminology and Criminal Justice
- Criminal Justice
- Criminology
- Forms of Crime
- International and Comparative Criminology
- Youth Violence and Juvenile Justice
- Development Studies
- Browse content in Economics
- Agricultural, Environmental, and Natural Resource Economics
- Asian Economics
- Behavioural Finance
- Behavioural Economics and Neuroeconomics
- Econometrics and Mathematical Economics
- Economic Systems
- Economic History
- Economic Methodology
- Economic Development and Growth
- Financial Markets
- Financial Institutions and Services
- General Economics and Teaching
- Health, Education, and Welfare
- History of Economic Thought
- International Economics
- Labour and Demographic Economics
- Law and Economics
- Macroeconomics and Monetary Economics
- Microeconomics
- Public Economics
- Urban, Rural, and Regional Economics
- Welfare Economics
- Browse content in Education
- Adult Education and Continuous Learning
- Care and Counselling of Students
- Early Childhood and Elementary Education
- Educational Equipment and Technology
- Educational Strategies and Policy
- Higher and Further Education
- Organization and Management of Education
- Philosophy and Theory of Education
- Schools Studies
- Secondary Education
- Teaching of a Specific Subject
- Teaching of Specific Groups and Special Educational Needs
- Teaching Skills and Techniques
- Browse content in Environment
- Applied Ecology (Social Science)
- Climate Change
- Conservation of the Environment (Social Science)
- Environmentalist Thought and Ideology (Social Science)
- Natural Disasters (Environment)
- Social Impact of Environmental Issues (Social Science)
- Browse content in Human Geography
- Cultural Geography
- Economic Geography
- Political Geography
- Browse content in Interdisciplinary Studies
- Communication Studies
- Museums, Libraries, and Information Sciences
- Browse content in Politics
- African Politics
- Asian Politics
- Chinese Politics
- Comparative Politics
- Conflict Politics
- Elections and Electoral Studies
- Environmental Politics
- European Union
- Foreign Policy
- Gender and Politics
- Human Rights and Politics
- Indian Politics
- International Relations
- International Organization (Politics)
- International Political Economy
- Irish Politics
- Latin American Politics
- Middle Eastern Politics
- Political Methodology
- Political Communication
- Political Philosophy
- Political Sociology
- Political Behaviour
- Political Economy
- Political Institutions
- Political Theory
- Politics and Law
- Politics of Development
- Public Administration
- Public Policy
- Quantitative Political Methodology
- Regional Political Studies
- Russian Politics
- Security Studies
- State and Local Government
- UK Politics
- US Politics
- Browse content in Regional and Area Studies
- African Studies
- Asian Studies
- East Asian Studies
- Japanese Studies
- Latin American Studies
- Middle Eastern Studies
- Native American Studies
- Scottish Studies
- Browse content in Research and Information
- Research Methods
- Browse content in Social Work
- Addictions and Substance Misuse
- Adoption and Fostering
- Care of the Elderly
- Child and Adolescent Social Work
- Couple and Family Social Work
- Direct Practice and Clinical Social Work
- Emergency Services
- Human Behaviour and the Social Environment
- International and Global Issues in Social Work
- Mental and Behavioural Health
- Social Justice and Human Rights
- Social Policy and Advocacy
- Social Work and Crime and Justice
- Social Work Macro Practice
- Social Work Practice Settings
- Social Work Research and Evidence-based Practice
- Welfare and Benefit Systems
- Browse content in Sociology
- Childhood Studies
- Community Development
- Comparative and Historical Sociology
- Economic Sociology
- Gender and Sexuality
- Gerontology and Ageing
- Health, Illness, and Medicine
- Marriage and the Family
- Migration Studies
- Occupations, Professions, and Work
- Organizations
- Population and Demography
- Race and Ethnicity
- Social Theory
- Social Movements and Social Change
- Social Research and Statistics
- Social Stratification, Inequality, and Mobility
- Sociology of Religion
- Sociology of Education
- Sport and Leisure
- Urban and Rural Studies
- Browse content in Warfare and Defence
- Defence Strategy, Planning, and Research
- Land Forces and Warfare
- Military Administration
- Military Life and Institutions
- Naval Forces and Warfare
- Other Warfare and Defence Issues
- Peace Studies and Conflict Resolution
- Weapons and Equipment

- < Previous chapter
- Next chapter >

48 Problem Solving
Department of Psychological and Brain Sciences, University of California, Santa Barbara
- Published: 03 June 2013
- Cite Icon Cite
- Permissions Icon Permissions
Problem solving refers to cognitive processing directed at achieving a goal when the problem solver does not initially know a solution method. A problem exists when someone has a goal but does not know how to achieve it. Problems can be classified as routine or nonroutine, and as well defined or ill defined. The major cognitive processes in problem solving are representing, planning, executing, and monitoring. The major kinds of knowledge required for problem solving are facts, concepts, procedures, strategies, and beliefs. Classic theoretical approaches to the study of problem solving are associationism, Gestalt, and information processing. Current issues and suggested future issues include decision making, intelligence and creativity, teaching of thinking skills, expert problem solving, analogical reasoning, mathematical and scientific thinking, everyday thinking, and the cognitive neuroscience of problem solving. Common themes concern the domain specificity of problem solving and a focus on problem solving in authentic contexts.
The study of problem solving begins with defining problem solving, problem, and problem types. This introduction to problem solving is rounded out with an examination of cognitive processes in problem solving, the role of knowledge in problem solving, and historical approaches to the study of problem solving.
Definition of Problem Solving
Problem solving refers to cognitive processing directed at achieving a goal for which the problem solver does not initially know a solution method. This definition consists of four major elements (Mayer, 1992 ; Mayer & Wittrock, 2006 ):
Cognitive —Problem solving occurs within the problem solver’s cognitive system and can only be inferred indirectly from the problem solver’s behavior (including biological changes, introspections, and actions during problem solving). Process —Problem solving involves mental computations in which some operation is applied to a mental representation, sometimes resulting in the creation of a new mental representation. Directed —Problem solving is aimed at achieving a goal. Personal —Problem solving depends on the existing knowledge of the problem solver so that what is a problem for one problem solver may not be a problem for someone who already knows a solution method.
The definition is broad enough to include a wide array of cognitive activities such as deciding which apartment to rent, figuring out how to use a cell phone interface, playing a game of chess, making a medical diagnosis, finding the answer to an arithmetic word problem, or writing a chapter for a handbook. Problem solving is pervasive in human life and is crucial for human survival. Although this chapter focuses on problem solving in humans, problem solving also occurs in nonhuman animals and in intelligent machines.
How is problem solving related to other forms of high-level cognition processing, such as thinking and reasoning? Thinking refers to cognitive processing in individuals but includes both directed thinking (which corresponds to the definition of problem solving) and undirected thinking such as daydreaming (which does not correspond to the definition of problem solving). Thus, problem solving is a type of thinking (i.e., directed thinking).
Reasoning refers to problem solving within specific classes of problems, such as deductive reasoning or inductive reasoning. In deductive reasoning, the reasoner is given premises and must derive a conclusion by applying the rules of logic. For example, given that “A is greater than B” and “B is greater than C,” a reasoner can conclude that “A is greater than C.” In inductive reasoning, the reasoner is given (or has experienced) a collection of examples or instances and must infer a rule. For example, given that X, C, and V are in the “yes” group and x, c, and v are in the “no” group, the reasoning may conclude that B is in “yes” group because it is in uppercase format. Thus, reasoning is a type of problem solving.
Definition of Problem
A problem occurs when someone has a goal but does not know to achieve it. This definition is consistent with how the Gestalt psychologist Karl Duncker ( 1945 , p. 1) defined a problem in his classic monograph, On Problem Solving : “A problem arises when a living creature has a goal but does not know how this goal is to be reached.” However, today researchers recognize that the definition should be extended to include problem solving by intelligent machines. This definition can be clarified using an information processing approach by noting that a problem occurs when a situation is in the given state, the problem solver wants the situation to be in the goal state, and there is no obvious way to move from the given state to the goal state (Newell & Simon, 1972 ). Accordingly, the three main elements in describing a problem are the given state (i.e., the current state of the situation), the goal state (i.e., the desired state of the situation), and the set of allowable operators (i.e., the actions the problem solver is allowed to take). The definition of “problem” is broad enough to include the situation confronting a physician who wishes to make a diagnosis on the basis of preliminary tests and a patient examination, as well as a beginning physics student trying to solve a complex physics problem.
Types of Problems
It is customary in the problem-solving literature to make a distinction between routine and nonroutine problems. Routine problems are problems that are so familiar to the problem solver that the problem solver knows a solution method. For example, for most adults, “What is 365 divided by 12?” is a routine problem because they already know the procedure for long division. Nonroutine problems are so unfamiliar to the problem solver that the problem solver does not know a solution method. For example, figuring out the best way to set up a funding campaign for a nonprofit charity is a nonroutine problem for most volunteers. Technically, routine problems do not meet the definition of problem because the problem solver has a goal but knows how to achieve it. Much research on problem solving has focused on routine problems, although most interesting problems in life are nonroutine.
Another customary distinction is between well-defined and ill-defined problems. Well-defined problems have a clearly specified given state, goal state, and legal operators. Examples include arithmetic computation problems or games such as checkers or tic-tac-toe. Ill-defined problems have a poorly specified given state, goal state, or legal operators, or a combination of poorly defined features. Examples include solving the problem of global warming or finding a life partner. Although, ill-defined problems are more challenging, much research in problem solving has focused on well-defined problems.
Cognitive Processes in Problem Solving
The process of problem solving can be broken down into two main phases: problem representation , in which the problem solver builds a mental representation of the problem situation, and problem solution , in which the problem solver works to produce a solution. The major subprocess in problem representation is representing , which involves building a situation model —that is, a mental representation of the situation described in the problem. The major subprocesses in problem solution are planning , which involves devising a plan for how to solve the problem; executing , which involves carrying out the plan; and monitoring , which involves evaluating and adjusting one’s problem solving.
For example, given an arithmetic word problem such as “Alice has three marbles. Sarah has two more marbles than Alice. How many marbles does Sarah have?” the process of representing involves building a situation model in which Alice has a set of marbles, there is set of marbles for the difference between the two girls, and Sarah has a set of marbles that consists of Alice’s marbles and the difference set. In the planning process, the problem solver sets a goal of adding 3 and 2. In the executing process, the problem solver carries out the computation, yielding an answer of 5. In the monitoring process, the problem solver looks over what was done and concludes that 5 is a reasonable answer. In most complex problem-solving episodes, the four cognitive processes may not occur in linear order, but rather may interact with one another. Although some research focuses mainly on the execution process, problem solvers may tend to have more difficulty with the processes of representing, planning, and monitoring.
Knowledge for Problem Solving
An important theme in problem-solving research is that problem-solving proficiency on any task depends on the learner’s knowledge (Anderson et al., 2001 ; Mayer, 1992 ). Five kinds of knowledge are as follows:
Facts —factual knowledge about the characteristics of elements in the world, such as “Sacramento is the capital of California” Concepts —conceptual knowledge, including categories, schemas, or models, such as knowing the difference between plants and animals or knowing how a battery works Procedures —procedural knowledge of step-by-step processes, such as how to carry out long-division computations Strategies —strategic knowledge of general methods such as breaking a problem into parts or thinking of a related problem Beliefs —attitudinal knowledge about how one’s cognitive processing works such as thinking, “I’m good at this”
Although some research focuses mainly on the role of facts and procedures in problem solving, complex problem solving also depends on the problem solver’s concepts, strategies, and beliefs (Mayer, 1992 ).
Historical Approaches to Problem Solving
Psychological research on problem solving began in the early 1900s, as an outgrowth of mental philosophy (Humphrey, 1963 ; Mandler & Mandler, 1964 ). Throughout the 20th century four theoretical approaches developed: early conceptions, associationism, Gestalt psychology, and information processing.
Early Conceptions
The start of psychology as a science can be set at 1879—the year Wilhelm Wundt opened the first world’s psychology laboratory in Leipzig, Germany, and sought to train the world’s first cohort of experimental psychologists. Instead of relying solely on philosophical speculations about how the human mind works, Wundt sought to apply the methods of experimental science to issues addressed in mental philosophy. His theoretical approach became structuralism —the analysis of consciousness into its basic elements.
Wundt’s main contribution to the study of problem solving, however, was to call for its banishment. According to Wundt, complex cognitive processing was too complicated to be studied by experimental methods, so “nothing can be discovered in such experiments” (Wundt, 1911/1973 ). Despite his admonishments, however, a group of his former students began studying thinking mainly in Wurzburg, Germany. Using the method of introspection, subjects were asked to describe their thought process as they solved word association problems, such as finding the superordinate of “newspaper” (e.g., an answer is “publication”). Although the Wurzburg group—as they came to be called—did not produce a new theoretical approach, they found empirical evidence that challenged some of the key assumptions of mental philosophy. For example, Aristotle had proclaimed that all thinking involves mental imagery, but the Wurzburg group was able to find empirical evidence for imageless thought .
Associationism
The first major theoretical approach to take hold in the scientific study of problem solving was associationism —the idea that the cognitive representations in the mind consist of ideas and links between them and that cognitive processing in the mind involves following a chain of associations from one idea to the next (Mandler & Mandler, 1964 ; Mayer, 1992 ). For example, in a classic study, E. L. Thorndike ( 1911 ) placed a hungry cat in what he called a puzzle box—a wooden crate in which pulling a loop of string that hung from overhead would open a trap door to allow the cat to escape to a bowl of food outside the crate. Thorndike placed the cat in the puzzle box once a day for several weeks. On the first day, the cat engaged in many extraneous behaviors such as pouncing against the wall, pushing its paws through the slats, and meowing, but on successive days the number of extraneous behaviors tended to decrease. Overall, the time required to get out of the puzzle box decreased over the course of the experiment, indicating the cat was learning how to escape.
Thorndike’s explanation for how the cat learned to solve the puzzle box problem is based on an associationist view: The cat begins with a habit family hierarchy —a set of potential responses (e.g., pouncing, thrusting, meowing, etc.) all associated with the same stimulus (i.e., being hungry and confined) and ordered in terms of strength of association. When placed in the puzzle box, the cat executes its strongest response (e.g., perhaps pouncing against the wall), but when it fails, the strength of the association is weakened, and so on for each unsuccessful action. Eventually, the cat gets down to what was initially a weak response—waving its paw in the air—but when that response leads to accidentally pulling the string and getting out, it is strengthened. Over the course of many trials, the ineffective responses become weak and the successful response becomes strong. Thorndike refers to this process as the law of effect : Responses that lead to dissatisfaction become less associated with the situation and responses that lead to satisfaction become more associated with the situation. According to Thorndike’s associationist view, solving a problem is simply a matter of trial and error and accidental success. A major challenge to assocationist theory concerns the nature of transfer—that is, where does a problem solver find a creative solution that has never been performed before? Associationist conceptions of cognition can be seen in current research, including neural networks, connectionist models, and parallel distributed processing models (Rogers & McClelland, 2004 ).
Gestalt Psychology
The Gestalt approach to problem solving developed in the 1930s and 1940s as a counterbalance to the associationist approach. According to the Gestalt approach, cognitive representations consist of coherent structures (rather than individual associations) and the cognitive process of problem solving involves building a coherent structure (rather than strengthening and weakening of associations). For example, in a classic study, Kohler ( 1925 ) placed a hungry ape in a play yard that contained several empty shipping crates and a banana attached overhead but out of reach. Based on observing the ape in this situation, Kohler noted that the ape did not randomly try responses until one worked—as suggested by Thorndike’s associationist view. Instead, the ape stood under the banana, looked up at it, looked at the crates, and then in a flash of insight stacked the crates under the bananas as a ladder, and walked up the steps in order to reach the banana.
According to Kohler, the ape experienced a sudden visual reorganization in which the elements in the situation fit together in a way to solve the problem; that is, the crates could become a ladder that reduces the distance to the banana. Kohler referred to the underlying mechanism as insight —literally seeing into the structure of the situation. A major challenge of Gestalt theory is its lack of precision; for example, naming a process (i.e., insight) is not the same as explaining how it works. Gestalt conceptions can be seen in modern research on mental models and schemas (Gentner & Stevens, 1983 ).
Information Processing
The information processing approach to problem solving developed in the 1960s and 1970s and was based on the influence of the computer metaphor—the idea that humans are processors of information (Mayer, 2009 ). According to the information processing approach, problem solving involves a series of mental computations—each of which consists of applying a process to a mental representation (such as comparing two elements to determine whether they differ).
In their classic book, Human Problem Solving , Newell and Simon ( 1972 ) proposed that problem solving involved a problem space and search heuristics . A problem space is a mental representation of the initial state of the problem, the goal state of the problem, and all possible intervening states (based on applying allowable operators). Search heuristics are strategies for moving through the problem space from the given to the goal state. Newell and Simon focused on means-ends analysis , in which the problem solver continually sets goals and finds moves to accomplish goals.
Newell and Simon used computer simulation as a research method to test their conception of human problem solving. First, they asked human problem solvers to think aloud as they solved various problems such as logic problems, chess, and cryptarithmetic problems. Then, based on an information processing analysis, Newell and Simon created computer programs that solved these problems. In comparing the solution behavior of humans and computers, they found high similarity, suggesting that the computer programs were solving problems using the same thought processes as humans.
An important advantage of the information processing approach is that problem solving can be described with great clarity—as a computer program. An important limitation of the information processing approach is that it is most useful for describing problem solving for well-defined problems rather than ill-defined problems. The information processing conception of cognition lives on as a keystone of today’s cognitive science (Mayer, 2009 ).
Classic Issues in Problem Solving
Three classic issues in research on problem solving concern the nature of transfer (suggested by the associationist approach), the nature of insight (suggested by the Gestalt approach), and the role of problem-solving heuristics (suggested by the information processing approach).
Transfer refers to the effects of prior learning on new learning (or new problem solving). Positive transfer occurs when learning A helps someone learn B. Negative transfer occurs when learning A hinders someone from learning B. Neutral transfer occurs when learning A has no effect on learning B. Positive transfer is a central goal of education, but research shows that people often do not transfer what they learned to solving problems in new contexts (Mayer, 1992 ; Singley & Anderson, 1989 ).
Three conceptions of the mechanisms underlying transfer are specific transfer , general transfer , and specific transfer of general principles . Specific transfer refers to the idea that learning A will help someone learn B only if A and B have specific elements in common. For example, learning Spanish may help someone learn Latin because some of the vocabulary words are similar and the verb conjugation rules are similar. General transfer refers to the idea that learning A can help someone learn B even they have nothing specifically in common but A helps improve the learner’s mind in general. For example, learning Latin may help people learn “proper habits of mind” so they are better able to learn completely unrelated subjects as well. Specific transfer of general principles is the idea that learning A will help someone learn B if the same general principle or solution method is required for both even if the specific elements are different.
In a classic study, Thorndike and Woodworth ( 1901 ) found that students who learned Latin did not subsequently learn bookkeeping any better than students who had not learned Latin. They interpreted this finding as evidence for specific transfer—learning A did not transfer to learning B because A and B did not have specific elements in common. Modern research on problem-solving transfer continues to show that people often do not demonstrate general transfer (Mayer, 1992 ). However, it is possible to teach people a general strategy for solving a problem, so that when they see a new problem in a different context they are able to apply the strategy to the new problem (Judd, 1908 ; Mayer, 2008 )—so there is also research support for the idea of specific transfer of general principles.
Insight refers to a change in a problem solver’s mind from not knowing how to solve a problem to knowing how to solve it (Mayer, 1995 ; Metcalfe & Wiebe, 1987 ). In short, where does the idea for a creative solution come from? A central goal of problem-solving research is to determine the mechanisms underlying insight.
The search for insight has led to five major (but not mutually exclusive) explanatory mechanisms—insight as completing a schema, insight as suddenly reorganizing visual information, insight as reformulation of a problem, insight as removing mental blocks, and insight as finding a problem analog (Mayer, 1995 ). Completing a schema is exemplified in a study by Selz (Fridja & de Groot, 1982 ), in which people were asked to think aloud as they solved word association problems such as “What is the superordinate for newspaper?” To solve the problem, people sometimes thought of a coordinate, such as “magazine,” and then searched for a superordinate category that subsumed both terms, such as “publication.” According to Selz, finding a solution involved building a schema that consisted of a superordinate and two subordinate categories.
Reorganizing visual information is reflected in Kohler’s ( 1925 ) study described in a previous section in which a hungry ape figured out how to stack boxes as a ladder to reach a banana hanging above. According to Kohler, the ape looked around the yard and found the solution in a flash of insight by mentally seeing how the parts could be rearranged to accomplish the goal.
Reformulating a problem is reflected in a classic study by Duncker ( 1945 ) in which people are asked to think aloud as they solve the tumor problem—how can you destroy a tumor in a patient without destroying surrounding healthy tissue by using rays that at sufficient intensity will destroy any tissue in their path? In analyzing the thinking-aloud protocols—that is, transcripts of what the problem solvers said—Duncker concluded that people reformulated the goal in various ways (e.g., avoid contact with healthy tissue, immunize healthy tissue, have ray be weak in healthy tissue) until they hit upon a productive formulation that led to the solution (i.e., concentrating many weak rays on the tumor).
Removing mental blocks is reflected in classic studies by Duncker ( 1945 ) in which solving a problem involved thinking of a novel use for an object, and by Luchins ( 1942 ) in which solving a problem involved not using a procedure that had worked well on previous problems. Finding a problem analog is reflected in classic research by Wertheimer ( 1959 ) in which learning to find the area of a parallelogram is supported by the insight that one could cut off the triangle on one side and place it on the other side to form a rectangle—so a parallelogram is really a rectangle in disguise. The search for insight along each of these five lines continues in current problem-solving research.
Heuristics are problem-solving strategies, that is, general approaches to how to solve problems. Newell and Simon ( 1972 ) suggested three general problem-solving heuristics for moving from a given state to a goal state: random trial and error , hill climbing , and means-ends analysis . Random trial and error involves randomly selecting a legal move and applying it to create a new problem state, and repeating that process until the goal state is reached. Random trial and error may work for simple problems but is not efficient for complex ones. Hill climbing involves selecting the legal move that moves the problem solver closer to the goal state. Hill climbing will not work for problems in which the problem solver must take a move that temporarily moves away from the goal as is required in many problems.
Means-ends analysis involves creating goals and seeking moves that can accomplish the goal. If a goal cannot be directly accomplished, a subgoal is created to remove one or more obstacles. Newell and Simon ( 1972 ) successfully used means-ends analysis as the search heuristic in a computer program aimed at general problem solving, that is, solving a diverse collection of problems. However, people may also use specific heuristics that are designed to work for specific problem-solving situations (Gigerenzer, Todd, & ABC Research Group, 1999 ; Kahneman & Tversky, 1984 ).
Current and Future Issues in Problem Solving
Eight current issues in problem solving involve decision making, intelligence and creativity, teaching of thinking skills, expert problem solving, analogical reasoning, mathematical and scientific problem solving, everyday thinking, and the cognitive neuroscience of problem solving.
Decision Making
Decision making refers to the cognitive processing involved in choosing between two or more alternatives (Baron, 2000 ; Markman & Medin, 2002 ). For example, a decision-making task may involve choosing between getting $240 for sure or having a 25% change of getting $1000. According to economic theories such as expected value theory, people should chose the second option, which is worth $250 (i.e., .25 x $1000) rather than the first option, which is worth $240 (1.00 x $240), but psychological research shows that most people prefer the first option (Kahneman & Tversky, 1984 ).
Research on decision making has generated three classes of theories (Markman & Medin, 2002 ): descriptive theories, such as prospect theory (Kahneman & Tversky), which are based on the ideas that people prefer to overweight the cost of a loss and tend to overestimate small probabilities; heuristic theories, which are based on the idea that people use a collection of short-cut strategies such as the availability heuristic (Gigerenzer et al., 1999 ; Kahneman & Tversky, 2000 ); and constructive theories, such as mental accounting (Kahneman & Tversky, 2000 ), in which people build a narrative to justify their choices to themselves. Future research is needed to examine decision making in more realistic settings.
Intelligence and Creativity
Although researchers do not have complete consensus on the definition of intelligence (Sternberg, 1990 ), it is reasonable to view intelligence as the ability to learn or adapt to new situations. Fluid intelligence refers to the potential to solve problems without any relevant knowledge, whereas crystallized intelligence refers to the potential to solve problems based on relevant prior knowledge (Sternberg & Gregorenko, 2003 ). As people gain more experience in a field, their problem-solving performance depends more on crystallized intelligence (i.e., domain knowledge) than on fluid intelligence (i.e., general ability) (Sternberg & Gregorenko, 2003 ). The ability to monitor and manage one’s cognitive processing during problem solving—which can be called metacognition —is an important aspect of intelligence (Sternberg, 1990 ). Research is needed to pinpoint the knowledge that is needed to support intelligent performance on problem-solving tasks.
Creativity refers to the ability to generate ideas that are original (i.e., other people do not think of the same idea) and functional (i.e., the idea works; Sternberg, 1999 ). Creativity is often measured using tests of divergent thinking —that is, generating as many solutions as possible for a problem (Guilford, 1967 ). For example, the uses test asks people to list as many uses as they can think of for a brick. Creativity is different from intelligence, and it is at the heart of creative problem solving—generating a novel solution to a problem that the problem solver has never seen before. An important research question concerns whether creative problem solving depends on specific knowledge or creativity ability in general.
Teaching of Thinking Skills
How can people learn to be better problem solvers? Mayer ( 2008 ) proposes four questions concerning teaching of thinking skills:
What to teach —Successful programs attempt to teach small component skills (such as how to generate and evaluate hypotheses) rather than improve the mind as a single monolithic skill (Covington, Crutchfield, Davies, & Olton, 1974 ). How to teach —Successful programs focus on modeling the process of problem solving rather than solely reinforcing the product of problem solving (Bloom & Broder, 1950 ). Where to teach —Successful programs teach problem-solving skills within the specific context they will be used rather than within a general course on how to solve problems (Nickerson, 1999 ). When to teach —Successful programs teaching higher order skills early rather than waiting until lower order skills are completely mastered (Tharp & Gallimore, 1988 ).
Overall, research on teaching of thinking skills points to the domain specificity of problem solving; that is, successful problem solving depends on the problem solver having domain knowledge that is relevant to the problem-solving task.
Expert Problem Solving
Research on expertise is concerned with differences between how experts and novices solve problems (Ericsson, Feltovich, & Hoffman, 2006 ). Expertise can be defined in terms of time (e.g., 10 years of concentrated experience in a field), performance (e.g., earning a perfect score on an assessment), or recognition (e.g., receiving a Nobel Prize or becoming Grand Master in chess). For example, in classic research conducted in the 1940s, de Groot ( 1965 ) found that chess experts did not have better general memory than chess novices, but they did have better domain-specific memory for the arrangement of chess pieces on the board. Chase and Simon ( 1973 ) replicated this result in a better controlled experiment. An explanation is that experts have developed schemas that allow them to chunk collections of pieces into a single configuration.
In another landmark study, Larkin et al. ( 1980 ) compared how experts (e.g., physics professors) and novices (e.g., first-year physics students) solved textbook physics problems about motion. Experts tended to work forward from the given information to the goal, whereas novices tended to work backward from the goal to the givens using a means-ends analysis strategy. Experts tended to store their knowledge in an integrated way, whereas novices tended to store their knowledge in isolated fragments. In another study, Chi, Feltovich, and Glaser ( 1981 ) found that experts tended to focus on the underlying physics concepts (such as conservation of energy), whereas novices tended to focus on the surface features of the problem (such as inclined planes or springs). Overall, research on expertise is useful in pinpointing what experts know that is different from what novices know. An important theme is that experts rely on domain-specific knowledge rather than solely general cognitive ability.
Analogical Reasoning
Analogical reasoning occurs when people solve one problem by using their knowledge about another problem (Holyoak, 2005 ). For example, suppose a problem solver learns how to solve a problem in one context using one solution method and then is given a problem in another context that requires the same solution method. In this case, the problem solver must recognize that the new problem has structural similarity to the old problem (i.e., it may be solved by the same method), even though they do not have surface similarity (i.e., the cover stories are different). Three steps in analogical reasoning are recognizing —seeing that a new problem is similar to a previously solved problem; abstracting —finding the general method used to solve the old problem; and mapping —using that general method to solve the new problem.
Research on analogical reasoning shows that people often do not recognize that a new problem can be solved by the same method as a previously solved problem (Holyoak, 2005 ). However, research also shows that successful analogical transfer to a new problem is more likely when the problem solver has experience with two old problems that have the same underlying structural features (i.e., they are solved by the same principle) but different surface features (i.e., they have different cover stories) (Holyoak, 2005 ). This finding is consistent with the idea of specific transfer of general principles as described in the section on “Transfer.”
Mathematical and Scientific Problem Solving
Research on mathematical problem solving suggests that five kinds of knowledge are needed to solve arithmetic word problems (Mayer, 2008 ):
Factual knowledge —knowledge about the characteristics of problem elements, such as knowing that there are 100 cents in a dollar Schematic knowledge —knowledge of problem types, such as being able to recognize time-rate-distance problems Strategic knowledge —knowledge of general methods, such as how to break a problem into parts Procedural knowledge —knowledge of processes, such as how to carry our arithmetic operations Attitudinal knowledge —beliefs about one’s mathematical problem-solving ability, such as thinking, “I am good at this”
People generally possess adequate procedural knowledge but may have difficulty in solving mathematics problems because they lack factual, schematic, strategic, or attitudinal knowledge (Mayer, 2008 ). Research is needed to pinpoint the role of domain knowledge in mathematical problem solving.
Research on scientific problem solving shows that people harbor misconceptions, such as believing that a force is needed to keep an object in motion (McCloskey, 1983 ). Learning to solve science problems involves conceptual change, in which the problem solver comes to recognize that previous conceptions are wrong (Mayer, 2008 ). Students can be taught to engage in scientific reasoning such as hypothesis testing through direct instruction in how to control for variables (Chen & Klahr, 1999 ). A central theme of research on scientific problem solving concerns the role of domain knowledge.
Everyday Thinking
Everyday thinking refers to problem solving in the context of one’s life outside of school. For example, children who are street vendors tend to use different procedures for solving arithmetic problems when they are working on the streets than when they are in school (Nunes, Schlieman, & Carraher, 1993 ). This line of research highlights the role of situated cognition —the idea that thinking always is shaped by the physical and social context in which it occurs (Robbins & Aydede, 2009 ). Research is needed to determine how people solve problems in authentic contexts.
Cognitive Neuroscience of Problem Solving
The cognitive neuroscience of problem solving is concerned with the brain activity that occurs during problem solving. For example, using fMRI brain imaging methodology, Goel ( 2005 ) found that people used the language areas of the brain to solve logical reasoning problems presented in sentences (e.g., “All dogs are pets…”) and used the spatial areas of the brain to solve logical reasoning problems presented in abstract letters (e.g., “All D are P…”). Cognitive neuroscience holds the potential to make unique contributions to the study of problem solving.
Problem solving has always been a topic at the fringe of cognitive psychology—too complicated to study intensively but too important to completely ignore. Problem solving—especially in realistic environments—is messy in comparison to studying elementary processes in cognition. The field remains fragmented in the sense that topics such as decision making, reasoning, intelligence, expertise, mathematical problem solving, everyday thinking, and the like are considered to be separate topics, each with its own separate literature. Yet some recurring themes are the role of domain-specific knowledge in problem solving and the advantages of studying problem solving in authentic contexts.
Future Directions
Some important issues for future research include the three classic issues examined in this chapter—the nature of problem-solving transfer (i.e., How are people able to use what they know about previous problem solving to help them in new problem solving?), the nature of insight (e.g., What is the mechanism by which a creative solution is constructed?), and heuristics (e.g., What are some teachable strategies for problem solving?). In addition, future research in problem solving should continue to pinpoint the role of domain-specific knowledge in problem solving, the nature of cognitive ability in problem solving, how to help people develop proficiency in solving problems, and how to provide aids for problem solving.
Anderson L. W. , Krathwohl D. R. , Airasian P. W. , Cruikshank K. A. , Mayer R. E. , Pintrich P. R. , Raths, J., & Wittrock M. C. ( 2001 ). A taxonomy for learning, teaching, and assessing: A revision of Bloom’s taxonomy of educational objectives. New York : Longman.
Baron J. ( 2000 ). Thinking and deciding (3rd ed.). New York : Cambridge University Press.
Google Scholar
Google Preview
Bloom B. S. , & Broder B. J. ( 1950 ). Problem-solving processes of college students: An exploratory investigation. Chicago : University of Chicago Press.
Chase W. G. , & Simon H. A. ( 1973 ). Perception in chess. Cognitive Psychology, 4, 55–81.
Chen Z. , & Klahr D. ( 1999 ). All other things being equal: Acquisition and transfer of the control of variable strategy . Child Development, 70, 1098–1120.
Chi M. T. H. , Feltovich P. J. , & Glaser R. ( 1981 ). Categorization and representation of physics problems by experts and novices. Cognitive Science, 5, 121–152.
Covington M. V. , Crutchfield R. S. , Davies L. B. , & Olton R. M. ( 1974 ). The productive thinking program. Columbus, OH : Merrill.
de Groot A. D. ( 1965 ). Thought and choice in chess. The Hague, The Netherlands : Mouton.
Duncker K. ( 1945 ). On problem solving. Psychological Monographs, 58 (3) (Whole No. 270).
Ericsson K. A. , Feltovich P. J. , & Hoffman R. R. (Eds.). ( 2006 ). The Cambridge handbook of expertise and expert performance. New York : Cambridge University Press.
Fridja N. H. , & de Groot A. D. ( 1982 ). Otto Selz: His contribution to psychology. The Hague, The Netherlands : Mouton.
Gentner D. , & Stevens A. L. (Eds.). ( 1983 ). Mental models. Hillsdale, NJ : Erlbaum.
Gigerenzer G. , Todd P. M. , & ABC Research Group (Eds.). ( 1999 ). Simple heuristics that make us smart. Oxford, England : Oxford University Press.
Goel V. ( 2005 ). Cognitive neuroscience of deductive reasoning. In K. J. Holyoak & R. G. Morrison (Eds.), The Cambridge handbook of thinking and reasoning (pp. 475–492). New York : Cambridge University Press.
Guilford J. P. ( 1967 ). The nature of human intelligence. New York : McGraw-Hill.
Holyoak K. J. ( 2005 ). Analogy. In K. J. Holyoak & R. G. Morrison (Eds.), The Cambridge handbook of thinking and reasoning (pp. 117–142). New York : Cambridge University Press.
Humphrey G. ( 1963 ). Thinking: An introduction to experimental psychology. New York : Wiley.
Judd C. H. ( 1908 ). The relation of special training and general intelligence. Educational Review, 36, 28–42.
Kahneman D. , & Tversky A. ( 1984 ). Choices, values, and frames. American Psychologist, 39, 341–350.
Kahneman D. , & Tversky A. (Eds.). ( 2000 ). Choices, values, and frames. New York : Cambridge University Press.
Kohler W. ( 1925 ). The mentality of apes. New York : Liveright.
Larkin J. H. , McDermott J. , Simon D. P. , & Simon H. A. ( 1980 ). Expert and novice performance in solving physics problems. Science, 208, 1335–1342.
Luchins A. ( 1942 ). Mechanization in problem solving. Psychological Monographs, 54 (6) (Whole No. 248).
Mandler J. M. , & Mandler G. ( 1964 ). Thinking from associationism to Gestalt. New York : Wiley.
Markman A. B. , & Medin D. L. ( 2002 ). Decision making. In D. Medin (Ed.), Stevens’ handbook of experimental psychology, Vol. 2. Memory and cognitive processes (2nd ed., pp. 413–466). New York : Wiley.
Mayer R. E. ( 1992 ). Thinking, problem solving, cognition (2nd ed). New York : Freeman.
Mayer R. E. ( 1995 ). The search for insight: Grappling with Gestalt psychology’s unanswered questions. In R. J. Sternberg & J. E. Davidson (Eds.), The nature of insight (pp. 3–32). Cambridge, MA : MIT Press.
Mayer R. E. ( 2008 ). Learning and instruction. Upper Saddle River, NJ : Merrill Prentice Hall.
Mayer R. E. ( 2009 ). Information processing. In T. L. Good (Ed.), 21st century education: A reference handbook (pp. 168–174). Thousand Oaks, CA : Sage.
Mayer R. E. , & Wittrock M. C. ( 2006 ). Problem solving. In P. A. Alexander & P. H. Winne (Eds.), Handbook of educational psychology (2nd ed., pp. 287–304). Mahwah, NJ : Erlbaum.
McCloskey M. ( 1983 ). Intuitive physics. Scientific American, 248 (4), 122–130.
Metcalfe J. , & Wiebe D. ( 1987 ). Intuition in insight and non-insight problem solving. Memory and Cognition, 15, 238–246.
Newell A. , & Simon H. A. ( 1972 ). Human problem solving. Englewood Cliffs, NJ : Prentice-Hall.
Nickerson R. S. ( 1999 ). Enhancing creativity. In R. J. Sternberg (Ed.), Handbook of creativity (pp. 392–430). New York : Cambridge University Press.
Nunes T. , Schliemann A. D. , & Carraher D. W , ( 1993 ). Street mathematics and school mathematics. Cambridge, England : Cambridge University Press.
Robbins P. , & Aydede M. (Eds.). ( 2009 ). The Cambridge handbook of situated cognition. New York : Cambridge University Press.
Rogers T. T. , & McClelland J. L. ( 2004 ). Semantic cognition: A parallel distributed processing approach. Cambridge, MA : MIT Press.
Singley M. K. , & Anderson J. R. ( 1989 ). The transfer of cognitive skill. Cambridge, MA : Harvard University Press.
Sternberg R. J. ( 1990 ). Metaphors of mind: Conceptions of the nature of intelligence. New York : Cambridge University Press.
Sternberg R. J. ( 1999 ). Handbook of creativity. New York : Cambridge University Press.
Sternberg R. J. , & Gregorenko E. L. (Eds.). ( 2003 ). The psychology of abilities, competencies, and expertise. New York : Cambridge University Press.
Tharp R. G. , & Gallimore R. ( 1988 ). Rousing minds to life: Teaching, learning, and schooling in social context. New York : Cambridge University Press.
Thorndike E. L. ( 1911 ). Animal intelligence. New York: Hafner.
Thorndike E. L. , & Woodworth R. S. ( 1901 ). The influence of improvement in one mental function upon the efficiency of other functions. Psychological Review, 8, 247–261.
Wertheimer M. ( 1959 ). Productive thinking. New York : Harper and Collins.
Wundt W. ( 1973 ). An introduction to experimental psychology. New York : Arno Press. (Original work published in 1911).
Further Reading
Baron, J. ( 2008 ). Thinking and deciding (4th ed). New York: Cambridge University Press.
Duncker, K. ( 1945 ). On problem solving. Psychological Monographs , 58(3) (Whole No. 270).
Holyoak, K. J. , & Morrison, R. G. ( 2005 ). The Cambridge handbook of thinking and reasoning . New York: Cambridge University Press.
Mayer, R. E. , & Wittrock, M. C. ( 2006 ). Problem solving. In P. A. Alexander & P. H. Winne (Eds.), Handbook of educational psychology (2nd ed., pp. 287–304). Mahwah, NJ: Erlbaum.
Sternberg, R. J. , & Ben-Zeev, T. ( 2001 ). Complex cognition: The psychology of human thought . New York: Oxford University Press.
Weisberg, R. W. ( 2006 ). Creativity . New York: Wiley.
- About Oxford Academic
- Publish journals with us
- University press partners
- What we publish
- New features
- Open access
- Institutional account management
- Rights and permissions
- Get help with access
- Accessibility
- Advertising
- Media enquiries
- Oxford University Press
- Oxford Languages
- University of Oxford
Oxford University Press is a department of the University of Oxford. It furthers the University's objective of excellence in research, scholarship, and education by publishing worldwide
- Copyright © 2024 Oxford University Press
- Cookie settings
- Cookie policy
- Privacy policy
- Legal notice
This Feature Is Available To Subscribers Only
Sign In or Create an Account
This PDF is available to Subscribers Only
For full access to this pdf, sign in to an existing account, or purchase an annual subscription.

Deciphering the Functions and Theories Behind Dreams
Brenda Jackson
Table of Contents
- Dreams have fascinated humans for centuries, with various theories and perspectives attempting to explain their meanings.
- Some theories, such as the activation-synthesis hypothesis and Freud’s theory of dream analysis, suggest that dreams are a product of random brain activity or symbolic representations of repressed desires and conflicts.
- Dreams serve multiple functions, including emotional processing, memory consolidation, preparation for potential threats, cognitive activation, and problem-solving.
- Dream interpretation is subjective and can vary based on personal beliefs, cultural factors, and individual experiences. Keeping a dream journal and reflecting on the content of dreams can provide insights into one’s unconscious mind and personal struggles.
The meaning of dreams has fascinated us for centuries, with many different theories existing about their meanings. While the true purpose of dreaming is not fully understood, we will explore different perspectives on dreams, including theories, processes, impacts, interpretations, and cultural and historical views.
Various Theories About Dreams
Dreams have fascinated and perplexed people for centuries. People have long sought to uncover the true meaning behind their dreams, believing that they offer insights into our subconscious minds and provide glimpses into the mysteries of our lives. There are several theories that attempt to explain why we dream and decipher the true significance of our dreams. While each theory offers a unique perspective on dream meanings, no single theory has been proven definitive. Let’s explore some of the most prominent theories below:
1. Activation-Synthesis Hypothesis
The activation-synthesis hypothesis suggests that dreams are simply the result of random brain activity during REM (rapid eye movement) sleep. According to this theory proposed by Harvard psychiatrists John Allan Hobson and Robert McCarley, our brains create stories from these random thoughts and images as a way to make sense of them. The content of our dreams is not significant and does not carry any hidden meanings, but rather it is our brain’s attempt to create a coherent narrative from the chaotic firing of neurons.
2. Freud’s Theory of Dream Analysis
Sigmund Freud, the father of psychoanalysis, believed that dreams were a window into the unconscious mind . According to Freud, dreams are symbolic representations of repressed desires, fears, and unresolved conflicts. He argued that the manifest content of a dream (the actual imagery and storyline ) is a disguise for the latent content (the hidden meaning). Freud believed that by analyzing dreams, one could gain insight into their true desires and conflicts , thus providing a pathway to self-discovery.
3. Threat Simulation Theory
The threat simulation theory suggests that dreams serve as a defense mechanism to prepare us for potential threatening events in waking life. This evolutionary-derived theory proposes that dreams allow us to practice cognitive mechanisms necessary for threat perception and avoidance . Through dream simulations, we can better prepare ourselves for potential dangers in reality.
4. Continual-Activation Theory
The continual-activation theory proposes that dreaming is a process by which our brains remain active during sleep to keep the mind functioning properly. This theory suggests that dreams serve as a way to maintain neural activation and keep the brain stimulated . While the exact purpose of this continual activation is not yet fully understood, some researchers believe it may contribute to memory consolidation and learning processes.
5. Other Theories
In addition to these prominent theories, there are several other theories about dream meanings. Some researchers propose that dreaming helps us process emotions and regulate our emotional experiences. Others suggest that dreams help us make sense of daily experiences and memories or serve as a creative outlet for problem-solving and idea generation.
It is important to note that these theories are not mutually exclusive, and dreams likely serve multiple functions based on individual experiences and circumstances. Additionally, the significance of dreams may vary from person to person, as personal beliefs and cultural factors can influence dream interpretation.
6. Making Sense of Your Dreams
While the true meaning of dreams remains elusive, many people find value in exploring their dream experiences. Keeping a dream journal and reflecting on the content of your dreams can help you uncover patterns, symbols, and emotions that may offer insights into your unconscious mind. By paying attention to your dreams and their connections to your waking life, you can gain a better understanding of yourself, your desires, and your challenges.
The Process and Impact of Dreaming
Dreaming is a fascinating and mysterious experience that occurs when we sleep. It involves vivid mental imagery, emotional shifts, and varying levels of awareness. But what exactly is the purpose and impact of dreaming? Let’s explore the process of dreaming and its effects on our emotional and cognitive wellbeing.
1. The Purpose of Dreams
Why do we dream? While scientists are still trying to fully understand the purpose of dreams, there are several theories that shed light on this phenomenon.
Emotional Processing
Dreams may serve as a way for our brains to process and regulate emotions. During REM sleep, which is when dreams are most vivid, our brains actively engage in emotional memory consolidation. This means that the brain sorts through the emotions we experienced during the day, deciding what to keep and what to let go. Dreams can help us process our feelings , making problems less daunting after a good night’s sleep.
Memory Consolidation
Another important function of dreams is memory consolidation. Our brains go through various stages of sleep throughout the night, and dreams can occur during both REM and non-REM sleep. During these different stages, our brains process and consolidate memories from the day. Dreams may help organize and integrate these memories, turning short-term memories into long-term ones.
Practice and Preparation
Some theories suggest that dreaming serves as a way for us to practice and prepare for real-life scenarios . Our early human ancestors faced daily threats to their survival, and dreams could have been a way for them to rehearse escaping danger or finding shelter. This practice in dreams may have prepared our ancestors to deal with similar situations in real life without any actual risk.
2. The Impact of Dreaming
Dreaming can have a significant impact on our sleep quality and overall wellbeing . Here are some ways in which dreams affect us:
Emotional Regulation
Dreaming provides an opportunity for emotional regulation . When we dream, our brains engage in emotional processing and can help defuse traumatic or distressing emotions. Dreams allow us to confront and work through feelings we might have suppressed during our waking hours, offering a safe space to explore and resolve emotional conflicts.
Cognitive Processing
Dreaming also supports cognitive processing , especially when it comes to memory consolidation. Dreams help organize and integrate information acquired during the day, turning it into long-term memories. This cognitive processing is vital for learning and memory formation .
Problem-Solving
Have you ever woken up with a solution to a problem or a new idea? Dreams have been known to inspire creativity and provide insights into difficult situations . They can offer new perspectives and innovative solutions by allowing us to think outside the box.
While not all dreams are pleasant, even nightmares play a role in our emotional and psychological wellbeing. Nightmares can be intense and distressing, but they can also serve as a way for us to confront fears, process traumatic experiences, and build resilience. Working through nightmares with the help of therapy or self-reflection can lead to emotional growth and healing .
Understanding and Interpreting Dreams
Dreams have fascinated and puzzled humans for centuries. We often wake up after a vivid dream and wonder if there is any deeper meaning behind it. While the true meaning of dreams remains a mystery , there are several theories and techniques that can help us understand and interpret them. In this section, we will explore some of the common theories of dream interpretation and provide tips for analyzing your own dreams.
1. Theories of Dream Interpretation
Freud’s theory of wish fulfillment.
Sigmund Freud, the renowned psychologist, believed that dreams are a manifestation of our unconscious desires . According to Freud, dreams serve as a way for our unconscious mind to fulfill our deepest wishes that may be repressed or unfulfilled in our waking life.
Jung’s Theory of Compensation
Carl Jung, another influential psychologist, proposed that dreams provide a balance or compensation for aspects of our personality that are in conflict with each other. He believed that dreams could reveal unseen parts of ourselves and offer insights into our psyche.
Hall’s Theory of Self-Reflection
Psychologist Calvin S. Hall suggested that dreams are a reflection of our thoughts and ideas. He believed that dreams could provide valuable insight into our sense of self, relationships, conflicts, and external environment.
Modern Theories
More recent theories on dream interpretation focus on the function and purpose of dreaming . Some scientists propose that dreams help with emotional processing, memory consolidation, performance improvement, and creativity enhancement.
2. Common Dream Themes
While the content of dreams can vary greatly from person to person, certain themes tend to recur in many people’s dreams. Here are some common dream topics and possible interpretations:
3. Tips for Analyzing and Interpreting Dreams
Analyzing and interpreting dreams can be a personal and introspective process. Here are some tips to help you understand the deeper meaning of your dreams:
- Keep a Dream Journal Record your dreams in a journal immediately after waking up. Write down as many details as you can remember, including emotions, people, objects, and locations.
- Look for Patterns and Symbols Pay attention to recurring patterns or symbols in your dreams. These may hold personal significance and can offer clues about underlying meanings.
- Consider Context and Personal Associations Reflect on the context of your dream and how it relates to your personal life. What was happening in your waking life before you had the dream? Are there any personal associations you make with the symbols or events in the dream?
- Seek Different Perspectives Share your dreams with trusted friends or family members and ask for their insights. Different perspectives can offer new insights and interpretations that you may not have considered.
- Consult with a Professional If you have recurring or troubling dreams that persistently impact your well-being, consider speaking with a mental health professional or dream analyst. They can help guide you through the interpretation process and provide support.
Remember that dream interpretation is subjective, and the meaning of your dream may be unique to you. Trust your intuition and allow yourself to explore different possibilities and interpretations.
Cultural and Historical Perspectives on Dreams
Dreams have always been a source of fascination and intrigue for humans throughout history. Different cultures and societies have developed their own interpretations and beliefs about the meaning and significance of dreams. Let’s explore some cultural and historical perspectives on dreams that shed light on this fascinating phenomenon .
1. Ancient Civilizations and Dreams
In ancient civilizations, dreams were often seen as messages from the gods or a medium for spiritual communication . For example, in ancient Egypt, dreams were believed to be important omens that could provide guidance and insight into the future. The Egyptians even had professional dream interpreters who would help decipher the hidden meanings behind dreams.
2. Freud and the Unconscious Mind
In the early 20th century, Sigmund Freud, the father of psychoanalysis, revolutionized the field of dream interpretation. Freud believed that dreams were a window to the unconscious mind and could reveal repressed desires, conflicts, and wishes. He introduced the concept of dream symbolism, suggesting that the images and events in dreams were representations of hidden thoughts and emotions.
3. Jung and Collective Unconscious
Another influential figure in dream psychology was Carl Jung, who believed that dreams could provide access to the collective unconscious, a shared repository of symbols, archetypes, and experiences. Jung saw dreams as a way to tap into universal themes and connect with the deeper aspects of human nature.
4. Cross-Cultural Perspectives
Dreams also vary across different cultures, reflecting their unique beliefs and traditions . In indigenous cultures, dreams often hold spiritual significance and are seen as a means of communication with ancestors or spirits. These cultures pay close attention to dreams, considering them valuable sources of wisdom and guidance .
In many African cultures, dreams are regarded as sources of healing or divination. They are believed to offer insights into illness or provide answers to important questions. Dream rituals and ceremonies are often performed to harness the power of dreams for spiritual or practical purposes.
5. Modern Perspectives on Dreams
Today, psychologists and anthropologists continue to study dreams and explore their cultural dimensions . Research has shown that dreams can be influenced by cultural practices, social experiences, and even political events.
Dreams have been found to reflect the collective anxieties and tensions within a society. For example, during times of social change or crisis, such as wars or revolutions, dreams may reflect the fears, hopes, and aspirations of individuals or communities. They can offer a unique perspective on the underlying social and psychological dynamics at play [1] during these transformative times.
6. Dream Interpretation Today
Dream interpretation remains a popular field of study and interest for many individuals. While there are no definitive answers to what dreams mean, exploring their cultural and historical significance can provide valuable insights into the human experience.
It’s important to remember that dream interpretation is subjective and personal. Dreams are deeply rooted in an individual’s unique experiences, emotions, and cultural background . What holds true for one person may not apply to another.
When it comes to interpreting your own dreams, it can be helpful to pay attention to the symbols, emotions, and themes that stand out. Keeping a dream journal and reflecting on the connections between your dreams and your waking life can provide valuable insights into your thoughts, feelings, and aspirations.
Ultimately, the real meaning of dreams is deeply personal and can vary from person to person. Exploring your dreams with an open mind and a curiosity about their cultural and historical dimensions can offer a richer understanding of yourself and the world around you.
Dreams remain a beautiful and mysterious aspect of our lives, providing glimpses into our innermost selves and offering us the chance to explore new possibilities. Whether you interpret them as mere products of random neural firings or as reflections of your deepest longings and fears, there is no denying the power and significance of our dreams. So next time you close your eyes and drift off to sleep, pay attention to the images that flit through your mind – who knows what treasures you might uncover? Remember to approach your dreams with curiosity and compassion, and you may find that they can offer you genuine insights into your life. Sweet dreams!
[1] The Cultural Dimensions of Dreaming
Related posts:
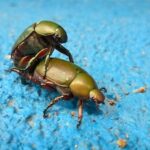
Leave a Reply Cancel reply
Your email address will not be published. Required fields are marked *
Save my name, email, and website in this browser for the next time I comment.

Recent Posts

- Animal Dreams 686
- Dream Science 50
- Dreams About Activities 350
- Dreams About Crystals 32
- Dreams About Food 214
- Dreams About Health 245
- Dreams About Me 182
- Dreams About People 297
- Dreams About Places 383
- Dreams About Plants 152
- Dreams About Things 552
- Dreams About Work 94
- Other Dreams 1,117
- Scary Dreams 282
- Supernatural Dreams 135
There’s no content to show here yet.

- Context and Narrative
- Dream Setting
- Dreamers Perspective
- Archetypal and Universal Themes
- Cultural and Symbolic References
- Dream Symbols
- Dreams and Creativity
- Dreams and Health
- Dreams and Memory Consolidation
- Interactions and Relationships
- Dreamers Actions and Choices
- Dreamers Feelings and Emotions
- Dreamers Feelings upon Awakening
- Cross-Cultural Dreaming
- Dreams in Society
- Meeting Famous People
- Social Dreaming Theory
- Lucidity and Control
- Personal Experiences
- Spiritual and Transcendent Dreams
- Applications and Implications
- Critiques and Controversies
- Dream Interpretation Techniques
- Overview and Historical Context
- Activation-Input-Modulation Model
- Activation-Synthesis Theory
- Cognitive Dream Theory
- Information-Processing Theory
- Neurocognitive Dream Theory
- Freudian Dream Theory
- Jungian Dream Theory
- Psychoanalytic Dream Theory
- Continual-Activation Theory
- Evolutionary Dream Theory
- Expectation Fulfillment Theory
- Problem-Solving Dream Theory
- Threat Simulation Theory
- Nightmares and Night Terrors
- Recurring Dreams
- Parallel Universe Dreams
- Past Life Dreams
- Telepathic Dreams
- Time-Travel Dreams
- Dreams Within Dreams
- Epic or Adventure Dreams
- False Awakenings
- Flying Dreams
- Lucid Dreams
- Fallen Angels
- Healing Dreams
- Prophetic Dreams
- Surreal Dreams
- Visitation Dreams
- Privacy Policy
- Terms and Conditions
- Mission Statement
Exploring Key Concepts of Cognitive Dream Theory

Have you ever wondered what drives the theories behind our dreams ? Cognitive Dream Theory suggests that our cognitive processes are at the heart of why we dream. By revealing the key concepts and principles of this fascinating theory , we can begin to understand the role that memory , information processing , and imagination play in constructing our dreams.
Does this theory hold the answers to the mysteries of our nightly visions? Delve deeper to uncover how cognitive functions intertwine with our dream worlds, leading you to a more profound comprehension of the mind ‘s nocturnal adventures.
Table of Contents
- 🧠 Interplay Between Cognition and Dreaming
- 📚 Overview of Cognitive Dream Theory
- 💤 Cognitive Dream Theory Processes in Dreams
- 🧩 Memory and Information Processing in Cognitive Dream Theory
- 🎨 Dream Construction and Imagination to Cognitive Dream Theory
- 📜 Cognitive Dream Theory Historical Development
- 👥 Notable Contributors to Cognitive Dream Theory
The Interplay Between Cognition and Dreaming
Have you ever pondered over the connection between your waking thoughts and the dreams you experience at night? Cognitive Dream Theory offers a compelling explanation, suggesting that our reasoning and problem-solving abilities don’t simply evaporate when we sleep. Instead, they shape the narratives and content of our dreams. This theory encompasses concepts such as memory consolidation , information processing , and the role of imagination in dream creation. But what exactly does this mean for you and the way you dream?
- Memory Consolidation: Dreams may help strengthen new memories.
- Information Processing: Our brains may sort and interpret daily experiences.
- Problem-Solving: Some theories suggest we work through issues in our dreams.
- Imagination: Dreams utilize our imaginative faculties to create scenarios.
- Emotional Regulation: Dreams could be a way our minds process emotions.
- Neural Activation: Dreaming might be linked to random brain activity during REM sleep.
- Cognitive Development: Dreams might reflect stages of cognitive development.
Interwoven Threads: Exploring the Cognitive Fabric of Dreamscapes
Before delving into these fascinating correlations, let’s consider how they interweave to generate the tapestry of our dreamscapes. These elements are like threads in a complex pattern, each contributing to the overall picture of our dream experiences.
The relationship between these cognitive processes and our dreams isn’t just fascinating—it’s illuminating. It offers a window into the ways our brains continue to work, even as we rest. These cognitive operations play a pivotal role in how we dream, what we dream about, and why certain dreams might be more memorable than others.
Cognitive Dream Theory weaves a narrative that connects our waking cognition to the stories told in sleep. Whether it’s the consolidation of memories or the subconscious processing of daily information, each aspect contributes to the understanding of our dream patterns. Embracing these insights can deepen our appreciation for the remarkable capabilities of the human mind.
An Overview of Cognitive Dream Theory
What is the essence behind the dreams we find ourselves immersed in during the night? Cognitive Dream Theory offers insights into this profound question, tying our dreaming experiences to the cognitive processes of our waking life. This theory sheds light on the intricate relationship between our thoughts , memories , and sensory experiences . It suggests that the way we learn, think, and perceive might directly influence the substance and structure of our dreams. Let’s unpack the foundational aspects of this thought-provoking theory.
- Cognitive Architecture: The mental frameworks that shape waking cognition also structure dreams.
- Consciousness Continuum: Dreams are seen as part of a continuum with waking conscious experience.
- Learning and Memory: Dreams may play a role in the consolidation and rehearsal of memories.
- Perceptual Processing: Sensory information from waking hours can be woven into the dream narrative.
- Reflection and Metacognition: Dreams might reflect one’s thoughts about thinking, or “thinking about thinking.”
Cognitive Sculptors: Understanding the Formation of Dream Experiences
Before we explore the table below, which will illuminate these concepts, it’s important to recognize how they collectively sculpt our dream experiences. Each cognitive component is a piece of the puzzle, contributing to the mental tapestry of our dreams. They hint at a deeper understanding of how and why our internal narratives unfold as they do in the dream world.
Cognitive Dream Theory posits a direct link between our waking cognitive experiences and the dreams we experience while asleep. It provides a framework to understand how dreams might function as extensions of our conscious life, involving similar cognitive processes like memory , learning , and perception . This theory encourages us to view dreams not as random nocturnal musings but as meaningful reflections of our cognitive self.
As we move from outlining the Overview of Cognitive Dream Theory , we’ve laid the groundwork to delve into the Key Concepts and Principles that underpin this fascinating framework. These principles are not standalone ideas but are deeply interwoven with the fabric of our dreaming minds. Moreover, they provide a scaffold for understanding how our cognitive processes manifest as we sleep. Now, let us turn our attention to these foundational elements—the building blocks that offer further clarity on the intricate dance between our cognition and the dreams it produces.
Cognitive Dream Theory Key Concepts and Principles
Diving into Cognitive Dream Theory reveals a realm where our waking life intricately intertwines with our dreams. The key concepts and principles of this theory underscore the continuity between our daily cognitive functions and nocturnal narratives. By understanding the roles of memory , learning , and consciousness , we gain insight into why we dream and what our dreams may signify. These principles serve as the guiding stars to navigate the intriguing landscape of our dreaming minds.
- Cognitive Continuity: Dreams are an extension of waking consciousness.
- Active Simulation: Dreams simulate experiences that could happen while awake.
- Memory Integration: Dreams integrate recent and past experiences.
- Emotional Processing: Dreams often reflect our emotional preoccupations.
- Thought Suppression: Suppressing thoughts by day may cause them to reemerge in dreams.
- Problem-Solving: Dreams may offer creative solutions to problems faced while awake.
- Mental Rehearsal: Practicing skills in dreams may improve waking performance.
Let’s connect these fundamental notions to see how they create the vibrant fabric of our dream experience. These concepts do not function in isolation but rather converge to form a dynamic cognitive dreamscape, enriching our understanding of the dreaming brain.
To encapsulate this section, the key concepts and principles of Cognitive Dream Theory present a comprehensive framework for understanding the intricate dance between our cognition and dream activity. Dreams are not just fantasies; they are the mind’s continuation of thought , emotion , and problem-solving from our waking hours. They offer a safe playground for the brain to explore scenarios, process memories , and even enhance our waking capabilities. This theory holds fascinating implications for the interconnectedness of our cognitive landscapes, whether we are asleep or awake.
Building upon our comprehension of the Key Concepts and Principles of Cognitive Dream Theory, we’ve encountered the intricate patterns that govern our dreamscapes. These principles are the bedrock upon which our understanding of dream cognition is built. Venturing forward, we prepare to explore the Processes in Dreams themselves—how our mental activities during the day continue to resonate through the night. This progression will illuminate the active role our brains play in shaping the dramas we experience in our sleep.
Cognitive Dream Theory Processes in Dreams
Within the enigmatic realm of our dreams, cognitive processes are actively at play. Cognitive Dream Theory posits that the same processes that guide our waking thought— perception , memory , learning , and reasoning —also manifest within our dreams. These processes are not random; rather, they are thought to be instrumental in the formation and progression of our dream narratives. But how do these cognitive processes manifest in our dreams, and what purpose might they serve?
- Perception: Dreams can involve complex sensory experiences.
- Memory: Elements from our waking life are interwoven into dreams.
- Learning: Dreams may help with problem-solving and integrating new knowledge.
- Emotional Regulation: Dreams often process and moderate our emotions.
- Creativity: They provide a rich tapestry for the expression of creative thought.
- Reflection: Dreams allow us to reflect on our sense of self and our beliefs.
- Language: Even in dreams, we often communicate and understand through language.
As we prepare to explore the structure of these cognitive operations , it’s essential to comprehend how they converge to mold our dream experiences. Each process contributes uniquely, offering a potential function or benefit to our waking lives. From memory consolidation to emotional processing, the complexity of dreams reflects the intricacies of our cognitive landscape.
These cognitive processes suggest that dreams are far more than mere echoes of our waking life; they are active rehearsals, creative explorations, and emotional safaris that serve pivotal functions in our cognitive and emotional well-being. They help us integrate new information, solve problems in innovative ways, and process emotions that we might not fully address during the day.
To encapsulate Cognitive Dream Theory’s view on dream processes, we can see dreams as a continuation of our cognitive functions into the night. They serve as a unique space where learning , memory integration , and emotional processing can occur without the constraints of reality. It’s in these nightly narratives that we can encounter the complexity of our cognitive powers, in all their vibrant and transformative glory.
Having explored the Processes in Dreams and how integral cognitive functions persist into our slumber, we’ve glimpsed the seamless continuity between waking and sleeping states. These processes provide a context for understanding the profound complexities of our dream experiences. Next, we’ll transition to a focused discussion on the Role of Memory and Information Processing in dreams, uncovering how these vital cognitive capacities affect the tapestry of our nocturnal narratives and contribute to our understanding of the mind’s inner workings.
Memory and Information Processing in Cognitive Dream Theory
In the intricate dance of dreams, memory and information processing play pivotal roles. According to Cognitive Dream Theory, dreams are not just random sequences; they are a reflection of the mind’s powerful ability to process, store, and recall information . By examining the function of memory within our dreams, we gain insights into how the brain uses the state of sleep to organize and manage the vast quantities of information we encounter daily. But what specific aspects of memory and information processing are at play within our dreams?
- Memory Consolidation: Dreams might aid in transferring information to long-term memory.
- Information Sorting: Our brains could be organizing daily experiences while we dream.
- Emotional Processing: Dreams often deal with emotionally charged memories.
- Problem Resolution: They can provide creative solutions that our waking minds didn’t see.
- Personal Reflection: Dreams may reflect our memories and thoughts about personal life events.
- Learning Integration: Dreams have a potential role in integrating new skills or knowledge.
- Nightly Rehearsals: They may serve as a stage for ‘rehearsing’ possible future scenarios.
In anticipation of a detailed view, let’s recognize the seamless integration of memory and information processing in our dream content. Each night, these cognitive functions collaborate to not just entertain but potentially improve our cognitive capacities during our waking hours.
Dreams reflect the brain’s nocturnal efforts to make sense of the day’s information glut. They appear to be not only a byproduct of our memory processes but also a contributing factor to them, playing a role in everything from emotional regulation to skill development . The night thus becomes an extension of our cognitive laboratory, where the synthesis of new knowledge and the refinement of memory are conducted beneath the veil of sleep.
Cognitive Dream Theory brings a greater appreciation for how dreams and memory intertwine. Dreams are not mere static reflections but dynamic and active rehearsals, highlighting the importance of memory consolidation and information processing in our internal nightly theater. With every dream, we potentially rehearse for life’s stage, wherein our memories and experiences are the script from which we perform.
We have delved into how Memory and Information Processing anchor our dreams to the realities of our daily lives, orchestrating the scenes that play out in the theater of the mind. These cognitive aspects not only store and sort our day’s experiences but also influence the emotional and narrative content of our dreams. Shifting our gaze forward, we will examine the Dream Construction and Imagination in Cognitive Dream Theory, where the boundless creativity of the mind sculpts our dream world, blending memory with the fantastical elements of imagination.
Dream Construction and Imagination to Cognitive Dream Theory
Imagine a world where your deepest thoughts, your most creative ideas, and your unexplored fantasies come to life—this is what happens every time we dream. Cognitive Dream Theory proposes that our imagination is not a passive bystander in this process; rather, it is a dynamic architect. It plays a fundamental role in the construction of dreams, leveraging the brain’s creative capacities to generate complex and vivid dreamscapes. But how exactly does imagination contribute to the way we dream, and what does this reveal about the cognitive processes at work?
- Imaginative Flexibility: Dreams reveal an uninhibited form of creativity.
- Scenario Simulation: They create intricate scenarios that simulate real or fantastical experiences.
- Emotional Exploration: Dreams allow for the safe expression of deep emotions.
- Cognitive Experimentation: They can be seen as a mental playground for new ideas.
- Problem-Solving: Dreams sometimes offer unconventional solutions to waking problems.
- Symbolism and Metaphor: They often employ symbols to represent complex thoughts or feelings.
- Sense of Wonder: Dreams can evoke awe and profound curiosity.
As we weave these threads together, we begin to see the vast tapestry of our dreams influenced by our imagination. They reflect the boundless potential of the mind to recreate and explore realities that extend beyond our waking life.
In dreaming, we discover the full scope of our creativity, untethered by the limits of reality. Dreams are a canvas where imagination paints scenes of extraordinary complexity, inviting us to question, explore, and even solve the riddles of our waking lives. They serve as a testament to the richness of our cognitive realm—where every night, we construct worlds that are as real in our minds as the waking world is to our senses.
The construct of dreams within the framework of Cognitive Dream Theory reveals the essence of imagination in our inner life. Dreams are not random; they are shaped by the very same forces that drive our creative endeavors when we are awake. They are proof that the human spirit, with its innate ability to dream, is fueled by an inexhaustible wellspring of inventiveness and insight . It’s through our dreams that we can tap into the profound depths of our imaginative potential.
As we’ve unraveled the role of Imagination in Dream Construction , we’ve seen the creative power of our minds at work, painting our sleep with vivid narratives and emotional landscapes. This intricate process of dream building emerges from a rich history of cognitive exploration. Now, we transition to examining the Historical Development of Cognitive Dream Theory , tracing the evolution of thought that has led to our current understanding of the dreaming mind. Through this historical lens, we gain perspective on the collective journey that has shaped the theories we study today.
Cognitive Dream Theory Historical Development
The evolution of Cognitive Dream Theory is as fascinating as the dreams it seeks to explain. This theory has not emerged in a vacuum; it stands on the shoulders of centuries of philosophical inquiry and decades of neuroscientific research . It represents a confluence of ideas that have been shaped by historical developments in understanding the human mind and its functions. How has Cognitive Dream Theory evolved over time, and what key milestones have influenced its current form?
- Ancient Philosophies: Early musings on the nature of dreams.
- Freudian Influence: The psychoanalytic perspective and its focus on dream symbolism.
- Behaviorism’s Impact: A shift towards observable behavior and away from mental processes.
- Cognitive Revolution: The resurgence of interest in internal mental states.
- Neuroscientific Advances: Modern technology unveiling the workings of the dreaming brain.
- Interdisciplinary Studies: Combining psychology, neuroscience, and other fields to understand dreams.
- Contemporary Theories: Ongoing research and theories that continue to shape our understanding.
As we prepare to chart the course of Cognitive Dream Theory’s historical progression, it’s crucial to appreciate the synergy of past and present thoughts that form the foundation of our current knowledge. Each phase in its evolution has contributed layers of understanding, constructing a comprehensive view of what dreams are and why they matter.
The historical development of Cognitive Dream Theory is a testament to the quest for understanding the hidden workings of the mind. Dreams, once the domain of myth and mysticism, have slowly unraveled to reveal their cognitive core through the contributions of numerous disciplines and epochs.
To encapsulate, Cognitive Dream Theory’s historical lineage showcases a journey from abstract musings to concrete scientific investigations. This evolution mirrors humanity’s own cognitive development, as we grow to understand the most intimate of experiences—our dreams. It’s a testament to the intertwined narrative of human thought and scientific discovery, how one begets the other, and how together, they illuminate the dark recesses of our night-time musings.
Reflecting on the Historical Development of Cognitive Dream Theory , we’ve witnessed a chronological tapestry unfold, revealing how our comprehension of dreams has been sculpted over time. This exploration into the past sets the stage for recognizing the individuals who have significantly shaped this field. Therefore, we segue into acknowledging the Notable Contributors to Cognitive Dream Theory , whose pioneering work and insightful discoveries continue to influence and inspire our quest to understand the enigmatic world of dreams.
Notable Contributors to Cognitive Dream Theory
The rich tapestry of Cognitive Dream Theory is embroidered with the insights of many brilliant minds. From early researchers to contemporary scholars, the contributions of these individuals have shaped our understanding of the cognitive mechanisms behind dreaming. These notable contributors have brought unique perspectives, innovative methodologies, and profound theories that underpin the study of dreams in the context of cognitive science. But who are these intellectual pioneers, and what have they bestowed upon the field?
- Sigmund Freud: The father of dream analysis and interpretation.
- Carl Jung: Emphasized the archetypal and collective unconscious in dreams.
- Calvin Hall: Focused on the cognitive aspects of dream content.
- J. Allan Hobson : Proposed the Activation-Synthesis Hypothesis of dreaming.
- Mark Solms : Integrated neuroscientific findings with psychoanalytic theories.
- G. William Domhoff: Advocated for a neurocognitive model of dreaming.
These contributors serve as beacons in the exploration of our nocturnal cognitive adventures, each illuminating a different facet of dreaming.
Through their groundbreaking work, these scholars have expanded the boundaries of how we comprehend the intricate connection between cognition and dreaming. They’ve provided frameworks, challenged existing paradigms, and opened doors to new realms of research.
Summarizing the contributions of these luminary figures, it becomes clear that Cognitive Dream Theory is a product of both divergent thinking and scholarly rigor. These theorists have given us a lens through which we can examine our dreams—not as mere figments of the imagination, but as complex cognitive phenomena. Their legacies continue to enlighten and inspire ongoing research, ensuring that the study of dreams remains a dynamic and ever-evolving field.
As we pull back the curtain on the mysteries of dreaming through the lens of Cognitive Dream Theory , it becomes apparent that our dreams are not mere byproducts of sleep but rather intricate expressions of our cognitive processes. From the fundamental principles that govern dream construction to the historical milestones that have shaped our understanding, we’ve navigated a realm where psychology, neurobiology, and philosophy converge. Notable contributors have paved the way for this exploration, each adding a unique piece to the dream puzzle.
Reflecting on this journey, one cannot help but marvel at the complex interplay between our waking cognition and the dreams that animate our nights. We’ve seen how memory, information processing, and imagination interweave to create the vivid tableau of our dreamscapes, and how the insights of Freud, Jung, and many others have advanced our understanding.
What do these insights mean for the future of dream research? As we stand at the crossroads of cognitive science and dream analysis, the path ahead promises even deeper insights into the architecture of the mind . We have only begun to chart the vast and unknown territories of our dreaming brains, and each night offers a new opportunity to explore the boundless potential of our consciousness.
With every dream, we are invited to question, to learn, and to wonder at the remarkable capabilities of our cognitive architecture —capabilities that do not sleep, even when we do. The ongoing conversation between our waking lives and our dreams is a testament to the unexplored depths of the human mind, a constant reminder that within each of us lies a world as vast and as mysterious as the universe itself.
- cognitive processes
- dream content
- dream recall
- emotional regulation
- memory consolidation
- neural networks
- problem-solving in dreams
- sleep stages
- Subconscious Mind

Related Articles

Cognitive Dream Theory: An Insightful Recap and Conclusion
This dream theory closes cognitive processes shaping our dreams, highlighting the importance...
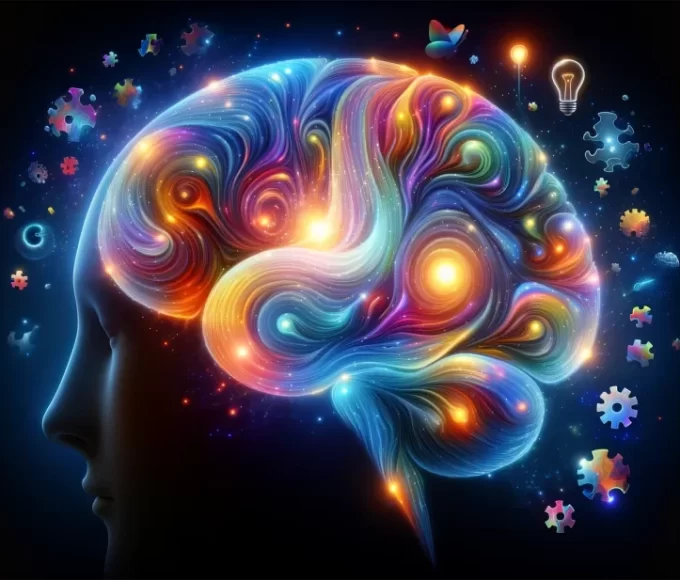
Exploring Cognitive Dream Theory: An Insightful Introduction
This dream interpretation guides you through the basics of cognitive dream theory,...
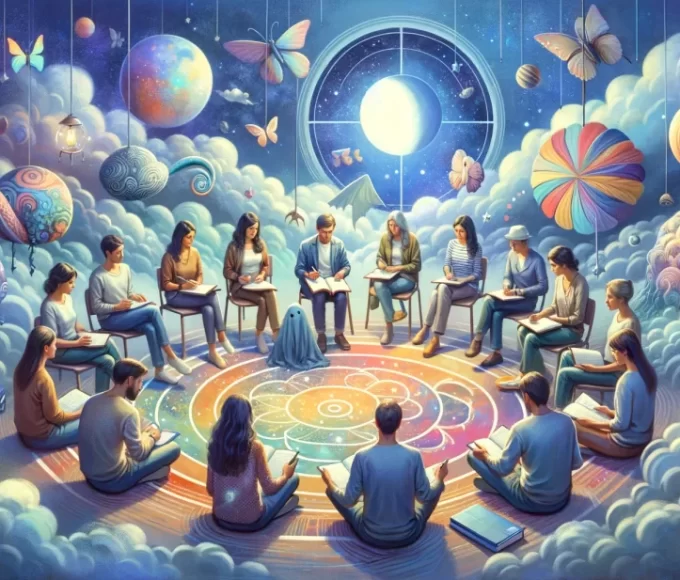
Cognitive Dream Theory: Bridging Theory and Practice
Explore the practical applications of cognitive dream theory in this dream theory,...

Cognitive Dream Theory: Insights from Research and Evidence
Explore cognitive dream theory through scientific studies, experiments, findings, and critiques in...
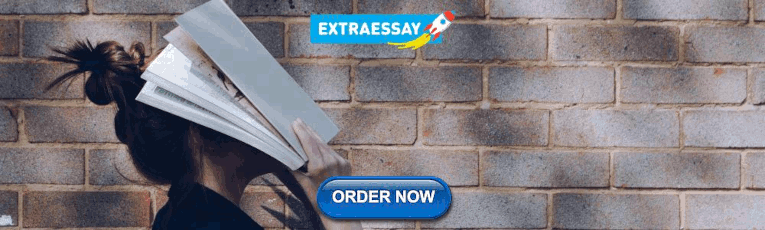
IMAGES
VIDEO
COMMENTS
Blagrove, M. (1992). Dreams as a reflection of our waking concerns and abilities: A critique of the problem-solving paradigm in dream research. Dreaming, 2, 205-220. Blagrove, M. (1996). Problems with the cognitive psychological modeling of dreaming. Journal of Mind and Behavior, 17, 99-134. Blagrove, M. (2000). Dreams have meaning but no function.
Dreams Images are the Embodiment of Thought. Central to Hall's cognitive theory is that dreams are thoughts displayed in the mind's private theater as visual concepts. Like Jung, Hall dismissed the Freudian notion that dreams are trying to cover something up. In his classic work The Meaning of Dreams (1966), Hall writes, "The images of a ...
Blagrove, M. (1992). Dreams as a reflection of our waking concerns and abilities: A critique of the problem-solving paradigm in dream research. Dreaming, 2, 205-220. Blagrove, M. (1996). Problems with the cognitive psychological modeling of dreaming. Journal of Mind and Behavior, 17, 99-134. Blagrove, M. (2000). Dreams have meaning but no function.
He added that Freud's dream theory was not scientific because it was not testable or falsifiable. ... In addition it provided new results suggesting that dreaming may have some psychological problem-solving function (this result recalls the neuroscientific findings that sleep has a cognitive problem-solving function associated with brain ...
The key cognitive process in dreaming is "simulation," a particular kind or subset of thinking that involves imaginatively placing oneself in a hypothetical scenario and ... theories. And the problem-solving theory of dream function has fewer and fewer adherents. Memory Consolidation? Social Rehearsal?
Within the context of current research in cognitive science, it is proposed that at least some dreams are generated by a regulatory system seeking to establish organismic balance, and in this sense fulfill a problem-solving function. ... Adler's Theory of Dreams: An Holistic Approach to Interpretation, in Handbook of Dreams, Wolman B. B ...
Considers methods of knowing dreams and what is meant by dream interpretation. Within the context of current research in cognitive science, it is proposed that at least some dreams are generated by a regulatory system seeking to establish organismic balance and, in this sense, fulfill a problem-solving function. A 5-step method designed to facilitate dream understanding is sketched: It is a ...
address the issue of the contribution of dreaming to the solution of problems / mention the main cognitive strategies involved in the solution of problems / review some data and opinions about cognitive abilities in dreaming / propose a general schema of the dream production mechanisms and deal with the processes of sequential organization, selection of meanings and memory retrieval / conclude ...
5. The psychodynamic dream generation model. Moser's dream generation model (Moser and von Zeppelin, 1996; Moser and Hortig, 2019) is based on psychodynamic dream theory, developmental and cognitive psychology, as well as experimental dream research.Moser et al. consider the sleep dream as a simulated micro-world controlled by affectivity, which generates images of entities involved in it and ...
With respect to the first group, the concept of "protoconsciousness" is the theory that at best explains lucid dreaming. With respect to theories with an evolutionary and adaptive function of dreams, those theories, that stress the problem solving or simulation functions of dreams are more suited to explain lucid dreaming.
Problem-Solving Theory Problem-cube Solved The problem-solving theory is a cognitive theory of dreaming that states the function of dreams is to help people solve their ongoing problems. In Cartwright's theory, dreams are a series of images activated by ongoing concerns, which are sought to be solved. Dreams help individuals solve ongoing problems
He also discusses the degree to which there is symbolism in dreams, the development of dreaming in children, and the relative frequency of emotions in the dreams of children and adults. During dreaming, the neural substrates that support waking sensory input, task-oriented thinking, and movement are relatively deactivated.
solving function (Blagrove, 1991, 1992a, 1992b, 1992c, dreaming, or the interpretation of dreams, is adaptive, will be problematic. Before the examination of cognitive models of empirical work on dream single-mindedness will first be reviewed. The Process of Dreaming - The Problem of Self Intention, and Control.
The Problem-Solving Theory of Dream Function 265 Emotions-Related Theories of the Adaptive Function of Dreaming 267 Mastery and Rehearsal Theories of Dream Function 273 Dreaming as a Culturally and Individually Useful By-Product 286 Conclusions and Implications 289 11 The Neurocognitive Theory Compared to Other Dream Theories 291
Problem Solving / physiology*. Dreams have produced art, music, novels, films, mathematical proofs, designs for architecture, telescopes, and computers. Dreaming is essentially our brain thinking in another neurophysiologic state-and therefore it is likely to solve some problems on which our waking minds have become stuck. This n ….
Lucid dreaming has clinical and scientific applications, and shows emerging potential as a methodology in the cognitive neuroscience of consciousness. Further research with larger sample sizes and refined methodology is needed. Keywords: lucid dreaming, consciousness, meta-awareness, dreaming, REM sleep. Go to:
distinguish cognitive operations that are quick and associative from others that are ... general label of dual-process theories (Chaiken and Trope, 1999; Hammond, 1996; Sloman, 1996). Dual-process models come in many 'flavors,' but all distinguish ... dreams contribute to problem-solving in science. Auguste von Kekule discovered the ring ...
At a Glance. There is no single dream theory that fully explains all of the aspects of why we dream. The most prominent theory is that dreams help us to process and consolidate information from the previous day. However, other theories have suggested that dreams are critical for emotional processing, creativity, and self-knowledge.
Dreams are thought to provide an evolutionary advantage because of their capacity to repeatedly simulate potential threatening events. This process enhances the neurocognitive mechanisms required for efficient threat perception and avoidance. The expectation-fulfillment theory posits that dreaming serves to discharge emotional arousals (however ...
Of these three, ego theory has had the greatest impact upon current psychoanalytic theorizing. What we should like to do in this paper is to bring dream theory within the context of ego psychology by defending the proposition that dreaming is a cognitive process. Before addressing ourselves to this theisis, let us define a dream.
Cognitive—Problem solving occurs within the problem solver's cognitive system and can only be inferred indirectly from the problem solver's behavior (including biological changes, introspections, and actions during problem solving).. Process—Problem solving involves mental computations in which some operation is applied to a mental representation, sometimes resulting in the creation of ...
Some theories, such as the activation-synthesis hypothesis and Freud's theory of dream analysis, suggest that dreams are a product of random brain activity or symbolic representations of repressed desires and conflicts. ... cognitive activation, and problem-solving. Dream interpretation is subjective and can vary based on personal beliefs ...
Cognitive Dream Theory posits a direct link between our waking cognitive experiences and the dreams we experience while asleep. It provides a framework to understand how dreams might function as extensions of our conscious life, involving similar cognitive processes like memory, learning, and perception.This theory encourages us to view dreams not as random nocturnal musings but as meaningful ...
Dreams, Sigmund Freud famously stated, are "the royal road to the unconscious." Psychoanalysts still believe that dream contents are unconscious manifestations of subconscious mental turmoil and that the examination and understanding of that content can help dreamers rid themselves of inner conflicts. Many of today's psychoanalysts take a more ...