Making the Case for Sustainable Urban Drainage Systems as a Nature-Based Solution to Urban Flooding
- Open Access
- First Online: 02 September 2017
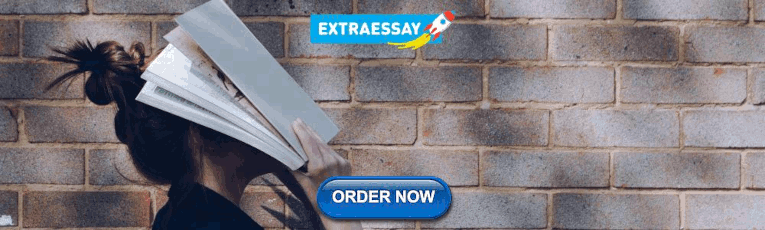
Cite this chapter
You have full access to this open access chapter
- McKenna Davis 10 &
- Sandra Naumann 10
Part of the book series: Theory and Practice of Urban Sustainability Transitions ((TPUST))
66k Accesses
31 Citations
5 Altmetric
European cities continue to experience a steady increase in the intensity and frequency of floods, largely due to high urban densities and resultant soil sealing. In the last decade, flooding as a natural hazard has produced the highest economic losses in Europe and storm water management has become a serious urban challenge.
The traditional solution to cope with excess rainwater in western cities has been piped drainage systems. These are mainly single-objective oriented designs that often no longer have the capacity to keep pace with on-going urbanisation and the impacts of climate change, and frequently involve high construction, maintenance, and repair costs. While such approaches have certainly reduced the damages incurred from flooding events during the past two centuries and are arguably still necessary for extreme flood events in the future, alternative approaches that accomplish these aims and offer additional benefits are progressively being pursued. Given these conditions, one increasingly utilised solution for managing flood risk by dealing with water at the source is sustainable urban drainage systems (SUDS). Other terms which also aim to minimise potential impacts on the neighbouring environment, people and development include inter alia BMP (Best Management Practices); LID (Low Impact Development); WSUD (Water Sensitive Urban Design) (see: Fletcher et al., Urban Water J 12(7):525–542, 2015 for a complete taxonomy).
SUDS as a promising nature-based solution are the focus of this chapter, utilizing a range of case studies and evidence from across Europe to underline the arguments presented. Besides reducing the negative effects of urban flooding and interlinked water pollution, the many supplementary benefits and potential cost-effectiveness of SUDS as compared to grey infrastructure solutions are also presented. In addition to highlighting relative advantages, the chapter also outlines current challenges facing a wider uptake of SUDS and presents approaches to help overcome existing social and political barriers.
The promise of ongoing research, targeted collaboration and partnerships and an ever-growing evidence base on the effectiveness and associated costs and benefits of SUDS serve as strong tools to improve the confidence and competence associated with their design and implementation. Such data will help to refute existing public and political hesitation as compares to traditional grey infrastructure approaches to water management. However, the significant potential for more widespread uptake remains largely untapped. Further targeted actions are necessary for increasing the acceptance and application of this nature-based solution and realizing its full potential.
You have full access to this open access chapter, Download chapter PDF
Similar content being viewed by others
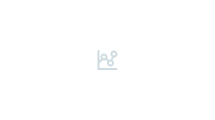
Sustainable urban drainage systems: examining the potential for green infrastructure-based stormwater management for Sub-Saharan cities
Nature-Oriented Paradigms for Urban Water Security: Perspective on Framework, Scale, and Sector
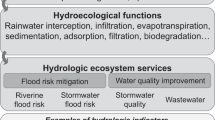
Blue–Green Infrastructure for Flood and Water Quality Management in Southeast Asia: Evidence and Knowledge Gaps
- Sustainable urban drainage system
- nature based solution
- flood management
- cost-benefit analysis
1 Introduction
European cities continue to experience an increase in the intensity and frequency of floods, with further escalations projected as a result of climate change and rapid urbanization (Santato et al. 2013 ). Particularly in urban areas, the management and drainage of storm water presents a serious challenge. The high urban density within cities and resultant soil sealing has lead to a reduction in the potential of water infiltration in the ground, which increases run-off water and flood risk (EEA 2012 ).
The traditional solution to these challenges in western cities has been ‘grey’ infrastructure – such as piped drainage systems – which are mainly single-objective oriented designs to cope with rainwater within the urban landscape. However, these drainage infrastructures often no longer have the capacity to keep pace with on-going urbanisation and the increasing rate of storm water due to climate change and soil sealing, and can lead to increased run-off and a higher risk of urban flooding (EEA 2012 ; Perales-Momparler et al. 2016 ; Zhou 2014 ). Additional indirect consequences are an insufficient discharge of excess water to the regional water system, and an increase of pollutants in the water caused by run-off (e.g., oil, organic matter and toxic metals), leading to increases in algal blooms, harm to wildlife and reductions in amenity value (Sharma 2008 ). Furthermore, managing storm water runoff through grey infrastructure approaches typically entails high construction, maintenance, and repair costs (Hair et al. 2014 ).
While ‘grey’ approaches have certainly reduced the damages incurred from flooding events during the past two centuries and are arguably still necessary for extreme flood events in the future, alternative approaches that accomplish these aims while offering additional benefits are progressively being pursued (Jones and Macdonald 2007 ; Perales-Momparler et al. 2016 ). Sustainable urban drainage systems (SUDS), Footnote 1 which are outlined in detail in the subsequent section, represent one such promising alternative flood risk management tool in the transition towards achieving regenerative urban built environments.
SUDS as a type of nature-based solution are the focus of this chapter, particularly concentrating on the existing evidence base regarding the potential for delivering a wide range of benefits as compares to purely grey solutions. The chapter also highlights challenges that are currently limiting more widespread uptake of these greener approaches and identifies potential solutions and needs to improve the confidence and competence associated with designing and implementing SUDS. The research results presented were gathered by the authors via an analysis of literature, expert interviews and EU level stakeholder workshops in the context of the EU research project RECREATE. Footnote 2
2 Using Green Alongside Grey as an Alternative Approach to Flood Protection
Instead of focusing on ‘end-of-pipe’ or ‘at the point of the problem’ solutions as is the case with many purely ‘grey’ infrastructure solutions, sustainable urban drainage systems aim to slow down and reduce the quantity of surface water runoff in an area in order to minimize downstream flood risk and reduce the risk of resultant diffuse pollution to urban water bodies (Rose and Lamond 2013 ; Woods Ballard et al. 2015 ; Zhou 2014 ). As a nature-based solution, SUDS achieves these aims by utilizing a mix of natural processes Footnote 3 and green/grey components Footnote 4 to harvest, infiltrate, slow, store, convey and treat runoff onsite; examples include the following (from Woods Ballard et al. 2015 ):
Rainwater harvesting systems – collect and store rainwater from roofs and other paved surfaces (such as car parks) for re-use
Green roofs – involve constructing a soil layer on a roof to create a living surface that reduces surface runoff
Permeable pavements – act as a hard surface for walking or driving, while enabling rainwater to infiltrate to the soil or underground storage
Bioretention systems (such as rain gardens) – collect runoff in a temporary surface pond before it filters through vegetation and underlying soils
Trees – capture rainwater while also providing evapotranspiration, biodiversity and shade
Swales, detention basins, retention ponds and wetlands – slow the flow of water, store and treat runoff while draining it through the site and encouraging biodiversity
Soakways and infiltration basins – promote infiltration as an effective means of controlling runoff and supporting groundwater recharge
These solutions are diverse in nature and can take many different forms both above and below ground, depending on the state and characteristics of the drainage system in place and the components utilized (State of Green 2015 ). Table 8.1 provides illustrative examples of different forms of SUDS which have been implemented across Europe.
SUDS can be implemented either as a new development or as a retrofit of existing structures. Regardless of the type, the central objective of all SUDS is to fully exploit the opportunities and benefits that can be obtained from surface water management (Woods Ballard et al. 2015 ).
3 Making the Investment Case for SUDS
In the past decade, more than 165 major floods evoking significant economic damages have taken place across Europe, making flooding (including from rivers, the sea and direct rainfall) the most widespread natural hazard on the continent in terms of economic loss (CRED 2009 ). In 2002, for example, flooding events occurred in six EU Member States and created infrastructure damages amounting to more than €18.7 billion (Santato et al. 2013 ). These damages highlight the shortcomings of piped systems as a stand-alone solution to address flooding, and imply the vast potential for replacing and complementing these systems with alternative nature-based approaches.
By integrating natural elements into their design, SUDS can serve to not only address the primary objective of improved water quality and quantity management, but can also offer a wide range of additional benefits which are supplementary to those of purely “grey” solutions (Charlesworth et al. 2016 ). SUDS can, for example, improve public health, create amenity values in the targeted areas, provide recreational opportunities, support the local ecology and biodiversity, and capture carbon (e.g., Burns et al. 2012 ; Charlesworth 2010 ; Norton et al. 2015 ; Novotny et al. 2010 ). Table 8.2 presents a more comprehensive overview of these and other potential benefits.
While the extent and nature of SUDS benefits are site-specific and depend on the attributes of the build or retrofit, several resources exist to support the quantification and monetisation of benefits offered in a given context. Such data serves to support decision-making processes and ultimately mainstream SUDS by providing comparative information on purely “grey” versus “green” or “mixed” solutions. The BeST tool (‘Benefits of SUDS Tool’), for example, was developed within the project ‘Demonstrating the multiple benefits of SUDS’ to enable practitioners to evaluate the wider benefits of SUDS in cases where surface water management is a key driver Footnote 5 (Digman et al. 2015 ). The UK’s SUDS Manual (see Woods Ballard et al. 2015 ) also outlines key concepts in estimating the costs and benefits of SUDS schemes and provides tools and further resources for assessments and comparisons to purely ‘grey’ infrastructure . A case study from this manual is provided in Box 8.1 , illustrating the potential benefits arising from a SUDS scheme versus a conventional drainage solution.
Box 8.1 Application of the BeST Tool to Compare the Benefits of Different Drainage Options: Roundhay Park, Leeds (UK)
Yorkshire Water utilized the BeST tool in order to compare the potential of different options to reduce combined sewer overflow (CSO) spills in Roundhay Park in Leeds (UK). As an additional decision-making criterion, the benefits that could be delivered by each option were also assessed (see Table 8.3 ). The four options considered used a range of conventional drainage and/or SUDS approaches, namely:
Option 1 : a conventional solution to store water in tanks at CSOs to limit the volume spilling
Option 2 : a conventional option also solving predicted flooding in the catchment, giving similar hydraulic performance in the combined sewer network as in options 3 and 4
Option 3 : a SUDS approach in public areas to disconnect surface water from the combined system and pass it through the conveyance and storage SUDS
Option 4 : as option 3, with measures added in residential private locations
Ultimately, Option 1 lowered the CSO spills but failed to generate other benefits. Option 2 would offer similar drainage benefits to the sewer network as Options 3 and 4 , but created less benefits due to underground infrastructure and was less resilient to climate change. As Options 3 and 4 created wider additional benefits to the community and environment with similar costs and benefits, the final selection was to pursue the public SUDS scheme as it had the best net present value ( Option 3 ). The associated costs and benefits are illustrated in Fig. 8.1 .
Comparison of options: costs vs benefits (Source: Woods Ballard et al. 2015 )
As shown in the above example, research also indicates that where SUDS “are designed to make efficient use of the space available, they can often cost less to implement than underground piped systems” (Woods Ballard et al. 2015 : 8) as well as less to maintain. Further comparative studies on the capital (and sometimes the maintenance) costs and benefits of traditional drainage and SUDS have been conducted by Defra as part of their work on the Flood and Water Management Act (see e.g., Defra 2011 , 2015 ). SUDS were found to offer cost savings of between approximately 10% and 85% as compared to traditional drainage approaches, with variations due to site and installation differences. Significant cost savings can be incurred inter alia due to the storage provided within landscape features and resultant reductions in the need for expensive boxed storage and creating low maintenance and monitoring costs (Defra 2011 ).
It should be emphasized, however, that further long-term research is necessary on the delivery and valuation of benefits as compares to piped solutions and particularly on the aspect of cost-effectiveness in different scenarios, contexts and combinations. Additional data is needed here to improve the targeted deployment of particular aspects and combinations of these technologies and design an optimal framework integrating technical, social, environmental, economic, legal and institutional aspects (Zhou 2014 ). These gaps as well as additional challenges to be addressed to foster a wider uptake of SUDS are outlined in the subsequent section.
4 Fostering a Wider Uptake and Implementation of SUDS
Despite the strong drivers and manifold benefits, SUDS have not been exploited or implemented to their full potential. Uncertainty about long-term maintenance, performance and (cost-) effectiveness both independently and as compared to purely grey infrastructure solutions serve as limitations to wider uptake. These informational limitations are particularly challenging to address as the restricted implementation in turn prohibits new data and evidence from being generated. Furthermore, the data and quantification of these aspects that do already exist are not widely known by the necessary actors, and therefore are commonly not considered alongside “grey” infrastructure. Given that SUDS are a rapidly evolving technology and are very site-specific in nature, another complication is that the levels of effectiveness, fulfilment of associated land requirements, costs and benefits vary greatly from case to case (Green Nylen and Kiparsky 2015 ). Technical, institutional/political, financial and social barriers relating to the above considerations are further impediments. Key challenges include obtaining the revenue to undertake maintenance, the potential land take and physical requirements involved in new developments, and the role of regulation (Ashley et al. 2015 ).
Strategies, regulatory frameworks and national level targets which exist to support SUDS implementation are currently scattered, with the majority of information on implementation and case studies limited to only a few countries (e.g. the UK, United States and Australia). For example, as a frontrunner in the field, the UK has SUDS legislation in place as part of the National Planning Policy Framework, which requires local authorities to include SUDS on new developments of 10 or more homes and all major new commercial and mixed use developments, unless demonstrated to be inappropriate. In consultation with the Lead Local Flood Authority, the Local Planning Authority then needs to approve drainage schemes (in line with non-statutory standards Footnote 6 ). Also in the UK, CIRIA Footnote 7 has published an extensive guidance manual addressing the planning, design, construction and maintenance of SUDS as well as tools for maximising amenity and biodiversity benefits alongside flood risk reduction and water quality improvement (see Woods Ballard et al. 2015 ).
However, even in the limited contexts where SUDS are comparatively more widely implemented, such as in the UK, the challenge remains to overcome silo thinking . SUDS are often raised as a possible approach only when targeting surface water and flooding issues, despite their potential to also address water quality challenges and deliver wider benefits in parallel. This stems in part from dispersed responsibilities amongst agencies for these topics as well as a problematic disconnect between research/development activities and implementation in many cases, leading to limited knowledge of available data and potential scepticism regarding its validity.
5 Addressing Silos and Informational Gaps
In order to address the outlined issues and improve the confidence and competence associated with designing and implementing SUDS, further coordinated research and targeted implementation initiatives are crucial. In addition to monitoring the performance, implementation and effectiveness of SUDS in cities, there is a need to disseminate this information highlighting the proven utility of SUDS in a targeted format to key stakeholder groups and decision makers. Such evidence could help appease existing hesitation and scepticism in choosing such a nature-based solution over the traditional grey alternative by providing evidence to questions of performance uncertainty. By providing a wealth of good practice experiences and accompanying monitoring data, a gradual change in stakeholder perception could be facilitated and therewith increased uptake and ecologic, socio-economic and monetary gains (Castro-Fresno et al. 2013 ; Perales-Momparler et al. 2016 ). Other research needs are on adequate institutional arrangements, human resource requirements, and performance indicators for urban drainage, which include the range of technical, economical, social and environmental aspects of SUDS (Ashley et al. 2013 ) as well as the improved quantification of benefits in order to capitalize on the potential future market.
Several research projects are aiming to fill these existing gaps by utilizing sound science to develop tools and guidance materials and implement demonstrative or pilot projects . The Danish 2BG “Black, Blue & Green” project, for example, commits to integrated infrastructure planning for sustainable urban water systems (DTU 2011 ), Ireland has several regional drainage assessment projects on integrated constructed wetlands, and the Swedish “Sustainable Urban Water Management” project focuses on protecting valuable water resources in urban areas. Footnote 8 The EU LIFE+ funded AQUAVAL project (“The efficient management of rain water in urban environments”) was highlighted earlier. It aims to find, implement and promote innovative solutions to decrease the impacts of developments on quantity and quality of urban runoff in Valencia, Spain, and implements SUDS as an important step in a paradigm shift (AQUAVAL 2010 ). Thames Water in the UK has also launched the ‘Twenty 4 Twenty’ initiative, a ca. 26 million Euro campaign aiming to transform at least 20 acres of grey impermeable concrete into sustainable drainage projects by 2020 (Thames Water 2015 ). Finally, a newly published study by Allitt et al. ( 2015 ) identifies the wider benefits of SUDS and provides guidance to water and sewerage companies on approaches to maximise the potential for benefits to be realised. The UK’s SUDS Manual (see Woods Ballard et al. 2015 ) also provides numerous good practice examples, tools and approaches for successful SUDS design, implementation and maintenance.
Due to the inherent need for cross-sectoral cooperation in designing, implementing and maintaining SUDS, efforts could also be placed on involving local communities in decision-making processes, instead of only presenting these actors with end results. This is underlined by Hair et al. ( 2014 ) and Ashley et al. ( 2013 ), who suggest to encourage stakeholder involvement and education at all levels of decision-making processes to improve transparency and foster trust and therewith increase acceptance and engagement by addressing citizen, business and political concerns (see Box 8.2 ). In this context, also employing collaborative governance approaches as suggested by Kabisch et al. ( 2016 ) to foster collaboration between decision makers and citizens, businesses and civil society connecting demands for action with responsible actors or partnerships for action could be a promising instrument to reduce barriers for adopting and implementing SUDS . Investments in social-cultural research and the development of cross-disciplinary language could be valuable venues by which to increase public acceptability and support, particularly given that many of the decisions on SUDS retrofits are the responsibility of property owners.
Box 8.2 Herne Hill and Dulwich Flood Alleviation Scheme : A Model for Citizen Engagement and Public-Private Cooperation in SUDS Implementation
Several linked SUDS were installed in a public and two private parks in Southwark, London (UK) in order to stop the recurrent flooding of homes and businesses along the River Effra. The award-winning scheme involved a public-private partnership and shared costs between Southwark Council and Thames Water, with support from the UK Environment Agency (EA). The scheme was designed and delivered in close collaboration and represents one of the first multi-agency SUDS schemes to be implemented in London. Of the total costs, the Council contributed 5%, Thames Water 54% and the EA mediated flood defence grant 41% in aid. Furthermore, Thames Water have provided funding to the Council for long-term maintenance, which is an important aspect for continued delivery given the 100-year design life of the project.
As a result of the project, 447 properties are at reduced risk of surface water flooding and over 80 properties have a reduced risk of sewer flooding. In addition to the direct economic benefits (valued at ca. 12 million pounds), the SUDS scheme has been praised for the extensive stakeholder involvement with the local community interest groups, businesses and residents. The invested outreach efforts were central to gaining support for the scheme and ensuring the continued delivery of amenity and environmental benefits. After receiving the ICE Engineering Award 2015, the EU Project Excellency Award 2015 (for partnership) and being shortlisted for the British Construction Industry Awards 2015, the SUDS project serves as a strong example for future approaches to reduceing surface water flooding risk in urban regions.
Source: Woods Ballard et al. ( 2015 )
Some initiatives have already been set in motion that support a more integrated approach or novel partnerships. For example, the Cooperative Research Centre for Water Sensitive Cities in Australia joins over 70 inter-disciplinary partners together to deliver sustainable water strategies that facilitate a city-wide transformation into a more liveable and resilient environment (CRC 2016 ). Further innovative approaches encouraging the implementation of SUDS and relevant green infrastructure elements by citizens were developed by the city of Hamburg, Germany. The RISA-project, Footnote 9 a cooperation between the city council and a private water company, aims to identify sustainable responses to avoid flooding of basements, streets and properties as well as water pollution from combined sewer overflow and urban run-off. It also seeks to integrate water management measures into urban and regional planning and develop a plan and guidance for rainwater management in the future. In addition, the city of Hamburg launched a green roof strategy Footnote 10 in 2016 providing financial support to citizens to install green roofs. In result not only water retention capacity in the city can be increased, but fees for sewage water and rainwater can also be reduced.
Efforts in the UK are also frontline in this regard, recognizing the value of partnerships to secure multiple sources of capital funding and share responsibility for long-term costs. The ‘Herne Hill and Dulwich flood alleviation scheme’, for instance, is an award-winning example of a successful public-private partnership for delivering SUDS (Southwark Council 2016 ; see Box 8.2 ). The UK Water Industry Research (UKWIR) Footnote 11 also finances proposals for collaborative research with joint funding and leverage, welcoming new partnerships and innovative associations for common research on SUDS.
6 Ways Forward for Increased SUDS Deployment
As urban populations grow alongside projected threats from climate change, demand is mounting for resilient future cities that can both protect the population from climatic events and offer further benefits in parallel. This chapter has thus presented a nature-based alternative to the historically pursued purely piped systems for addressing urban flooding which has the potential to support sustainable urban developments and provide recreational, aesthetic, environmental and socio-economic benefits. SUDS offer significant potential in this regard as evidence indicates a high potential for being sustainable, cost-effective approaches which can complement pure grey infrastructure , and can be applied within new developments or used to retrofit existing systems.
While a range of challenges have been outlined which threaten the wider uptake of SUDS, the promise of ongoing research, targeted collaborative and dissemination initiatives and an ever-growing evidence base of the effectiveness and associated costs/benefits of SUDS serve as strong countermeasures. Here, it is important to make lessons learned and data gathered from existing cases more widely available. New pilot or demonstration projects should also be promoted and invested in which are collaborative in nature and strengthen the links between researchers, practitioners and relevant community stakeholders. Finally, the targeted involvement of groups that are perhaps not traditionally interested in drainage matters, such as those in the health or transport sectors, and encouragement of exchanges between companies having implemented SUDS and those pursuing purely grey solutions can also benefit the mainstreaming of SUDS.
These efforts can in turn strengthen the ‘business case’ for SUDS by instilling more confidence in and drawing attention to their wider benefits produced, low comparative associated costs, and climate change compatible nature. Such evidence will help to refute public and political hesitation as compares to traditional grey infrastructure approaches to water management. Highlighting the delivery of the multiple benefits produced in addition to flood protection which traditional engineered flood protection schemes cannot deliver is a central element. New business-models for public-private partnerships are a further aspect of this process, combining blue/green spaces, human well-being, water management and climate change adaptation interests (see Box 8.2 for an example). Establishing such ‘business case’ arguments will serve as the foundation for increased investment, public and political support and ultimately SUDS deployment.
Once confidence exists that SUDS are effective and affordable as a nature-based solution technology, governments can increasingly support wider implementation. Means to do so include establishing an adequate legal framework that builds upon the evidence gathered and – alongside financial agreements/investment banks – helping to bridge the gap between short-term thinking and long-term investments via intentional regulatory design. At the EU level and in other industrialized countries, potential actions could experiment with and adjust institutional settings, considering alternative local capacities and site-specific cultural aspects. By requiring use of the technology and establishing duties for adoption and maintenance, governments can ensure the implementation of SUDS and facilitate a transition to becoming a ‘business as usual’ option and highlight the importance of such an approach as a national priority. More specifically, national regulators can use their authority to more actively accelerate and improve SUDS development by adopting standardized monitoring and reporting protocols and guidance and by incentivising and highlighting the importance of voluntary monitoring.
A strong evidence base exists which demonstrates the effectiveness of SUDS and highlights their promise as a sustainable solution to reduce urban flooding. Yet, the significant potential for more widespread uptake remains largely untapped. Further targeted actions are necessary for increasing the acceptance and application of this nature-based solution and realizing its full potential.
Other terms are used elsewhere: inter alia BMP (Best management practices); LID (Low Impact Development); WSUD (Water Sensitive Urban Design) (see: Fletcher et al. 2015 for a complete taxonomy)
REsearch network for forward looking activities and assessment of research and innovation prospects in the fields of Climate, Resource Efficiency and raw mATErials (RECREATE): URL: http://www.recreate-net.eu /
These could include, for example, evaporation, infiltration, re-use and plant transpiration.
Including, for example, permeable surfaces, filter strips, filter and infiltration trenches, green roofs, swales, detention basins, underground storage, wetlands and/or retention ponds.
See http://www.susdrain.org/resources/best.html
See https://www.gov.uk/government/uploads/system/uploads/attachment_data/file/415773/sustainable-drainage-technical-standards.pdf
CIRIA is a neutral, independent and not-for-profit British construction industry research and information association (see http://www.ciria.org /).
See http://www.urbanwater.se/en
http://www.risa-hamburg.de/english.html
http://www.hamburg.de/gruendach/4364756/gruendachfoerderung/
See https://www.ukwir.org /
2BG (2008) Sustainable urban drainage systems – 8 case studies from the Netherlands. Working Paper, University of Copenhagen & Technical University of Denmark, Black, Blue and Green PhD course, pp 20–22
Google Scholar
Allitt R, Tanzir C, Massie A, Sherrington CA (2015) Realising the wider benefits of sustainable drainage. ISBN: 184057 769 X
AQUAVAL (2010) Project Objectives. Accessed on 30 Sept 2016 at http://www.aquavalproject.eu/adaptingContenidos/muestrausuario.asp?accADesplegar=113&IdNodo=14
Ashley R, Shaffer P, Walker L (2013) Sustainable drainage systems: research roadmap. On behalf of UKWIR. Final report
Ashley RM, Walker L, D’Arcy B, Wilson S, Illman S, Shaffer P, Woods-Ballard B, Chatfield P (2015) UK sustainable drainage systems: past, present and future. Civil Engineering – Proceedings of the Institution of Civil Engineers. ICE Publishing
Burns et al (2012) Hydrologic shortcomings of conventional urban stormwater management and opportunities for reform. Landsc Urban Plan 105:230–240
Article Google Scholar
Castro-Fresno et al (2013) Sustainable drainage practicies in Spain, specially focused on pervious pavements. Water 5:67–93
Charlesworth (2010) A review of the adaptation and mitigation of global climate change using sustainable drainage in cities. J Water Clim Change 1(3):165–180
Charlesworth et al (2016) Renewable energy combined with sustainable drainage: ground source heat and pervious paving. Renewable and Sustainable Energy Reviews, Articile in Press, pp 1–8
CRC (2016) Global partnerships. Available online at: https://watersensitivecities.org.au/collaborate/global-partnerships/
CRED (2009) Disaster data: a balanced perspective. CRED Crunch, Centre for Research on the Epidemiology of Disasters (CRED), Issue No. 17, Brussels
Defra (2011) Comparative costings for surface water sewers and SUDS. Cases: Daniels Cross, Newport, Shropshire; Red Hill C.of E. Primary School, Worcester; Caledonian Road Housing, Islington, London; Railfreight Terminal, Telford, Shropshire; Marlborough Road, Telford, Shropshire; URL: http://www.susdrain.org/delivering-suds/using-suds/the-costs-and-benefits-of-suds/comparison-of-costs-and-benefits.html
Defra (2015) Cost estimation for SUDS – summary of evidence. Report –SC080039/R9
Digman CJ, Horton B, Ashley RM et al (2015) Getting the BeST from SUDS. In: Proceedings of the Chartered Institution of water and environmental management urban drainage group spring conference, Birmingham, UK
DTU (2011). 2BG: black, blue and green – Integrated infrastructure planning as key to sustainable urban water systems. Accessed 30 Sept 2016 at http://orbit.dtu.dk/en/projects/2bg-black-blue-and-green--integrated-infrastructure-planning-as-key-to-sustainable-urban-water-systems(36195120-7c7e-4efe-bc00-78d01bb7a678).html
EEA (2012) Urban adaptation to climate change in Europe – challenges and opportunities for cities together with supportive national and European policies
Fletcher T, Shuster W, Hunt WF, Ashley R, Butler D, Arthur S, Trowsdale S, Barraud S, Semadeni-Davies A, Bertrand-Krajewski J, Mikkelsen PS, Rivard G, Uhl M, Dagenais D, Viklander M (2015) SUDS, LID, BMPs, WSUD and more – the evolution and application of terminology surrounding urban drainage. Urban Water J 12(7):525–542
Green Nylen N, Kiparsky M (2015) Accelerating cost-effective green stormwater infrastructure: learning from local implementation. Center for Law, Energy & the Environment, U.C. Bekeley School of Law. http://law.berkeley.edu/cost-effective-GSI.htm
Hair L, Clements J, Pratt J (2014) Insights on the economics of green infrastructure: a case study approach. Water Economics Federation, WEFTEC 2014, pp 5556–5585
Jones P, Macdonald N (2007) Making space for unruly water: sustainable drainage systems and the disciplining of surface runoff. Geoforum 38:534–544
Kabisch N, Frantzeskaki N, Pauleit S, Naumann S, Davis M et al (2016) Nature-based solutions to climate change mitigation and adaptation in urban areas –perspectives on indicators, knowledge gaps, barriers and opportunities for action. Ecol Soc 21(2):39
Norton et al (2015) Planning for cooler cities: a framework to prioritise green infrastructure to mitigate high temperatures in urban landscapes. Landsc Urban Plan 135:127–138
Novotny et al (2010) Water centric sustainable communities: planning, retrofitting, and building the next Urban environment. Wiley, New Jersey. ISBN 978-0-470-47608-6
Book Google Scholar
Perales-Momparler S (2012) Demonstration SUDS in the Mediterranean region of Valencia. SUDSnet International Conference. 5th September 2012, Conventry University, UK. Available for download at: http://sudsnet.abertay.ac.uk/presentations/National%20Conf%202012/Session7_AQUAVALDemonstrationSUDSInTheMediterraneanRegionOfValencia_Perales.pdf
Perales-Momparler S, Andrés-Doménech I, Hernández-Crespo C, Vallés-Morán F, Martín M et al (2016) The role of monitoring sustainable drainage systems for promoting transition towards regenerative urban built environments: a case study in the Valencian region, Spain. J Clean Prod , Article in Press, pp 1–12
Pledger S (n.d.) Lamb Drove, Residential SUDS scheme, Cambourne. Accessed at http://www.susdrain.org/case-studies/case_studies/lamb_drove_residential_suds_scheme_cambourne.html , on 18 Sept 2016
Rose C, Lamond J (2013) Performance of sustainable drainage for urban flood control, lessons from Europe and Asia. In: International conference on flood resilience, experiences in Asia and Europe, Exeter, United Kingdom, 5–7 Sept 2013
Santato S, Bender S, Schaller M (2013)The European floods directive and opportunities offered by land use planning. CSC Report 12, Climate Service Center, Germany. Available for download at: http://www.climate-service-center.de/imperia/md/content/csc/csc-report_12.pdf
Sharma D (2008). Sustainable drainage system (SUDS) for stormwater management: a technological and policy intervention to combat diffuse pollution. 11th International conference on urban drainage, Edinburgh, Scotland, UK, 2008. Available for download at: http://web.sbe.hw.ac.uk/staffprofiles/bdgsa/11th_International_Conference_on_Urban_Drainage_CD/ICUD08/pdfs/753.pdf
Southwark Council (2016) Herne Hill and Dulwich Flood Alleviation Scheme. Accessed 30 Sept 2016 at http://www.southwark.gov.uk/info/200448/flood_risk_management/3889/herne_hill_and_dulwich_flood_alleviation_scheme
State of Green (2015) Sustainable urban drainage systems: using rainwater as a resource to create resilient and liveable cities. Available online at: https://stateofgreen.com/files/download/8247
Thames Water (2015) Twenty 4 twenty: sustianable drainage. Accessed on 30 Sept 2016 at http://www.thameswater.co.uk/about-us/19122.htm
Woods Ballard W, Wilson S, Udale-Clarke H, Illman S, Scott T, Ashley R, Kellagher R (2015) The SUDS Manual. CIRIA C697, London, 2015
Zhou Q (2014) A review of sustainable urban drainage systems considering the climate change and urbanization impacts. Water 6:976–992
Download references
Author information
Authors and affiliations.
Ecologic Institute, Berlin, Germany
McKenna Davis & Sandra Naumann
You can also search for this author in PubMed Google Scholar
Corresponding author
Correspondence to McKenna Davis .
Editor information
Editors and affiliations.
Helmholtz-Centre for Environmental Research – UFZ, Leipzig, Germany
Nadja Kabisch
Federal Agency of Nature Conservation (BfN), Isle of Vilm, Germany
Jutta Stadler
Friedrich Schiller University Jena, Jena, Germany
Aletta Bonn
Rights and permissions
Open Access This chapter is licensed under the terms of the Creative Commons Attribution 4.0 International License ( http://creativecommons.org/licenses/by/4.0/ ), which permits use, sharing, adaptation, distribution and reproduction in any medium or format, as long as you give appropriate credit to the original author(s) and the source, provide a link to the Creative Commons license and indicate if changes were made.
The images or other third party material in this chapter are included in the chapter’s Creative Commons license, unless indicated otherwise in a credit line to the material. If material is not included in the chapter’s Creative Commons license and your intended use is not permitted by statutory regulation or exceeds the permitted use, you will need to obtain permission directly from the copyright holder.
Reprints and permissions
Copyright information
© 2017 The Author(s)
About this chapter
Davis, M., Naumann, S. (2017). Making the Case for Sustainable Urban Drainage Systems as a Nature-Based Solution to Urban Flooding. In: Kabisch, N., Korn, H., Stadler, J., Bonn, A. (eds) Nature-Based Solutions to Climate Change Adaptation in Urban Areas. Theory and Practice of Urban Sustainability Transitions. Springer, Cham. https://doi.org/10.1007/978-3-319-56091-5_8
Download citation
DOI : https://doi.org/10.1007/978-3-319-56091-5_8
Published : 02 September 2017
Publisher Name : Springer, Cham
Print ISBN : 978-3-319-53750-4
Online ISBN : 978-3-319-56091-5
eBook Packages : Earth and Environmental Science Earth and Environmental Science (R0)
Share this chapter
Anyone you share the following link with will be able to read this content:
Sorry, a shareable link is not currently available for this article.
Provided by the Springer Nature SharedIt content-sharing initiative
- Publish with us
Policies and ethics
- Find a journal
- Track your research
Simulation-Based Optimisation of Sustainable Urban Drainage Systems
- Omid Seyedashraf
- Department of Mechanical, Aerospace & Civil Engineering
Student thesis : Phd
File : application/pdf, -1 bytes
Type : Thesis
- Help & FAQ
The effectiveness of certain sustainable urban drainage systems in controlling flooding and pollution from urban runoff
- Kirsteen Macdonald
Student thesis : Doctoral Thesis
File : application/pdf, 129 MB
Type : Thesis
Related content
Research output, assessing the performance of urban bmps in scotland.
Research output : Contribution to journal › Article › peer-review
We use cookies to provide you with the best user experience and to improve our services. Cookies help us understand how our site is being used, allowing us to enhance your browsing experience. By clicking "Accept," you consent to the use of all cookies. If you choose to reject, we’ll only use necessary cookies to ensure the site functions properly. Learn more .
Sustainable urban drainage systems (SUDS) MSc by Research, MPhil, PhD
Course detail, award title.
This is a potential research degree area, subject to the approval of the University. If you are interested in undertaking a research degree in this area, please get in touch to discuss your proposal.
The Engineering Division is home to the University’s leading specialist centre - the Urban Water Technology Centre (UWTC) that has a unique focus on the interface between scientific and technological research addressing the challenges of building resilient and sustainable communities. Our strengths derive from valuable links established between academia and industry at a national and international level in the construction and civil engineering, water, waste, manufacturing, food and drinks sectors.
Apply technological and sustainable solutions to contemporary problems
Our research focuses on applying technological and sustainable solutions to contemporary problems. Our knowledge transfer expertise and management supporting tools assist a wide range of industrial and non-industrial stakeholders such as national and local government, a wide range of private sector organisations, regulatory bodies, water and waste companies, Non-governmental organisations (NGOs) and health boards.
We have a formidable track record in combining academic skills and commercial expertise with our research approach being very much multi-disciplinary and industry-facing resulting in the expertise of our staff being much sought after by businesses and professionals in the field operating at an International Level. We are a member of SAGES network, a collaboration of Scottish Universities whose goal it is to provide the highest quality/world-leading postgraduate education and research experience.
What does a research degree involve?
As a postgraduate research student, you will carry out an original, independent research and/or practice-based project, whilst being guided and supported by a team of expert academic supervisors. As you do this, you will benefit from an extensive programme of researcher development tailored to your background, needs and future ambitions. This will in turn help you develop the skills required to deliver research excellence.
Your progress will be monitored throughout the year and via an annual review. The final assessment is by examination of your submitted thesis and a viva voce (oral examination).
With the help of your supervisors, you will be encouraged to publish your research during and after your studies.
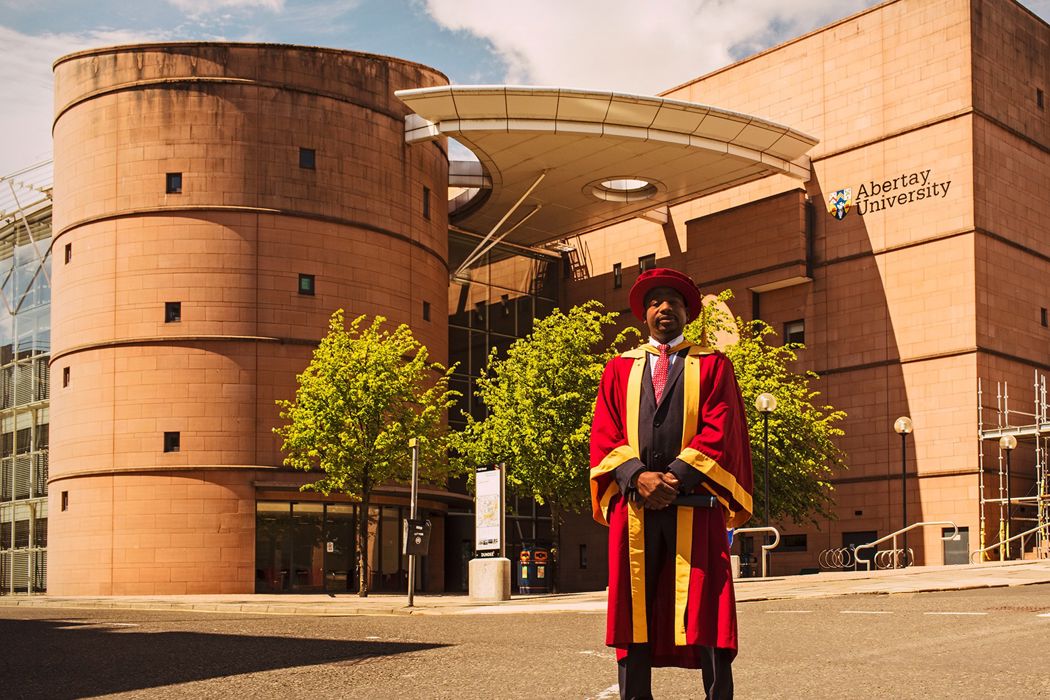
Our research degrees
You have the option of studying an MSc by Research, an MPhil or a PhD.
When deciding which degree is right for you, you should consider the level of commitment required, the duration, cost and your career aspirations.
That being said, our research degrees are flexible. You can begin an MSc or an MPhil and then seek to transfer to a PhD without having to start your project all over again. You can also switch from full-time to part-time study, and vice versa.
MSc by Research
The award of Master of Science by Research (MSc by Research) demonstrates a practical ability to undertake research studies, a systematic understanding of knowledge and a critical awareness of current problems and/or the application of original thought.
How long is an MSc by Research?
If you are studying full-time, you will be expected to complete the award in 12 months. The minimum period to receive this award is 10 months and the maximum is 14 months.
If you are studying part-time, you will be expected to complete the award in 24 months. The minimum period to receive this award on a part-time basis is 20 months and the maximum is 28 months.
The award of Master of Philosophy demonstrates a systematic understanding of knowledge and a critical awareness of current problems and/or the application of original thought, much of which is at (or informed by) the forefront of your field of study or area of professional practice.
How long is an MPhil?
If you are studying full-time, you are expected to complete the award in 24 months. The minimum period to receive this award is 12 months and the maximum is 48 months.
If you are studying part-time, you are expected to complete the award in 48 months. The minimum period to receive this award on a part-time basis is 24 months and the maximum is 72 months.
The award of the Doctor of Philosophy demonstrates that the main focus of your work is your personal contribution to knowledge in your discipline or field, through original research or the original application of existing knowledge.
How long is a PhD?
If you are studying full-time, you are expected to complete the award in 36 months. The minimum period to receive this is award is 24 months and the maximum is 48 months.
If you are studying part-time, you are expected to complete the award in 48 months. The minimum period to receive this award on a part-time basis is 72 months and the maximum is 96 months.
What makes Abertay's Graduate School different?
Abertay is a small university, which means that as a research student, you’ll be joining a close-knit community of scholars and researchers.
Watch Prof Nia White, Dean of Research and Abertay’s Graduate School, along with some of our research students discuss what makes Abertay’s Graduate School different from other universities.
Learn more about our Graduate School➟
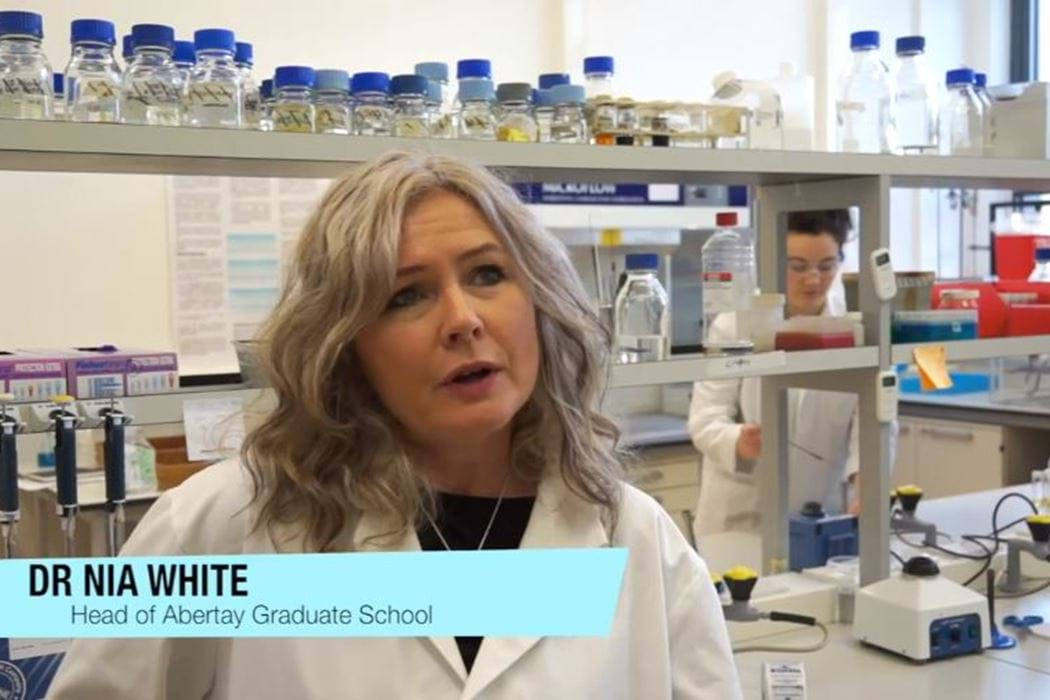
Fees and funding
The fees you'll pay depend on factors such as your nationality, location and personal circumstances. See the links below and scroll down to find the Postgraduate section.
Postgraduate student fees - Scottish Students
Postgraduate student fees - UK Students (outside Scotland)
Postgraduate student fees - International & EU Students
More information
Find out about potential bursaries, student loans and scholarships in our postgraduate research fees and funding section.
How to apply
1. meet the academic entry requirements.
The minimum entry requirement for all our research degrees is an Upper Second Class Honours degree (or equivalent) at undergraduate level in an appropriate discipline and/or a Master’s degree. In some cases, appropriate professional or experiential learning may be considered in combination with a lower classification of Honours degree.
2. Meet the visa and English language requirements
Visa requirements.
If you're not from the European Economic Area (EEA) and/or Switzerland, you may need to apply for a visa. You can find out more about applying for a visa and collecting your Biometric Residence Permit (BRP) on our Tier 4 visa page. To identify whether or not you need to apply for a visa, please visit the UKBA website.
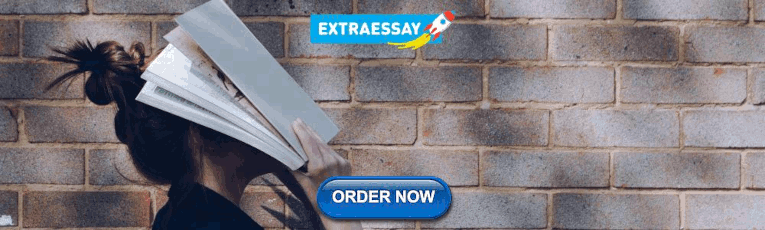
English language requirements
We accept an undergraduate or postgraduate degree that has been taught and assessed in a majority English speaking country, as defined by UK Visas and Immigration:
UKVI list of majority English speaking countries
For candidates whose first language is not English or your undergraduate/Master’s degree was not awarded from a University from a UKVI designated English Speaking country, you are required to show evidence of one of the following qualifications:
IELTS Academic: total 6.5 with at least 6.0 in each component.
TOEFL-iBT (including Home Edition): total 92 with at least 20 in each component. We do not accept TOEFL “My Best Score” to meet our English language requirements.
CAE and CPE: total 176 with at least 169 in each component.
Trinity ISE: ISE II with distinctions in all four components.
PTE Academic: 62 overall with at least 59 in each component.
NECO English at B (4)
WAEC English at B (4)
If you are not a national of a majority English speaking country, then your degree must be no more than three and a half years old at the beginning of your programme of study, unless you are using IELTS, TOEFL, Trinity ISE or PTE, in which case it must be no more than two years old.
To identify whether or not you need to apply for a student visa, please see here or visit the UKVI website.
3. Find a supervisor
You can look for potential supervisors by searching keywords, names or publications in the Staff Explorer . T his will ensure that we have appropriate expertise within Abertay. You will also gain an understanding of the prior work of the researcher.
You should consider contacting the potential supervisor to discuss your ideas and the possibility of undertaking a research degree under their supervision. Discussion will also help you tailor your application to suit Abertay’s specific expertise in the area. Ask for feedback and be prepared to take those comments into consideration when finalising your research proposal.
You can also contact the Dean of School or our Graduate School if you would like advice on potential supervisors.
Please avoid vague blanket emails to several potential supervisors as these are unlikely to be successful.
4. Secure funding
There are two different routes to studying a research degree at Abertay – you can apply for one of our funded studentships or propose your own area of research. Find out more about our funded studentships .
If you are proposing your own area of research, you will need to consider how you will fund your studies – either through self-support or by securing a scholarship or some other form of external funding. See what scholarships are available to Abertay research students .
5. Write your research proposal
This is your opportunity to state your research objectives, to grab the reader’s attention and highlight your suitability for research degree study.
The proposal should be around 6-8 pages in length (including references) and follow the section headings below.
Abstract (summary).
Introduction/background.
Hypotheses, objectives or research question.
Proposed methodology.
How will you disseminate your findings (pathways to impact).
Ethical considerations.
Summary and conclusions.
References.
About you: briefly describe your relevant experience and how it will help you achieve your objectives, explain why you are an excellent candidate for a research degree, and identify your additional professional development and training needs.
Some good practice tips:
Be clear and concise.
Structure your proposal by breaking up blocks of text into smaller paragraphs (with headings).
Reference you work.
Justify your objectives.
6. Apply online by the relevant deadline
You can start a research degree at Abertay in February, June or October of each year. You can apply to defer from one entry point to another on one occasion, however, subsequent requests must be supported by an additional full application.
Unless otherwise stated, the deadlines for submitting a full application are:
Applications for February entry by 15 December (if you need a Tier 4 visa then the deadline is 30 November).
Applications for June entry by 30 April (if you need a Tier 4 visa then the deadline is 31 March).
Applications for October entry by 31 August (if you need a Tier 4 visa then the deadline is 31 July).
You will need to include the following documentation when you apply online:
Copies of your academic transcripts
Your research proposal
Apply now to start in:
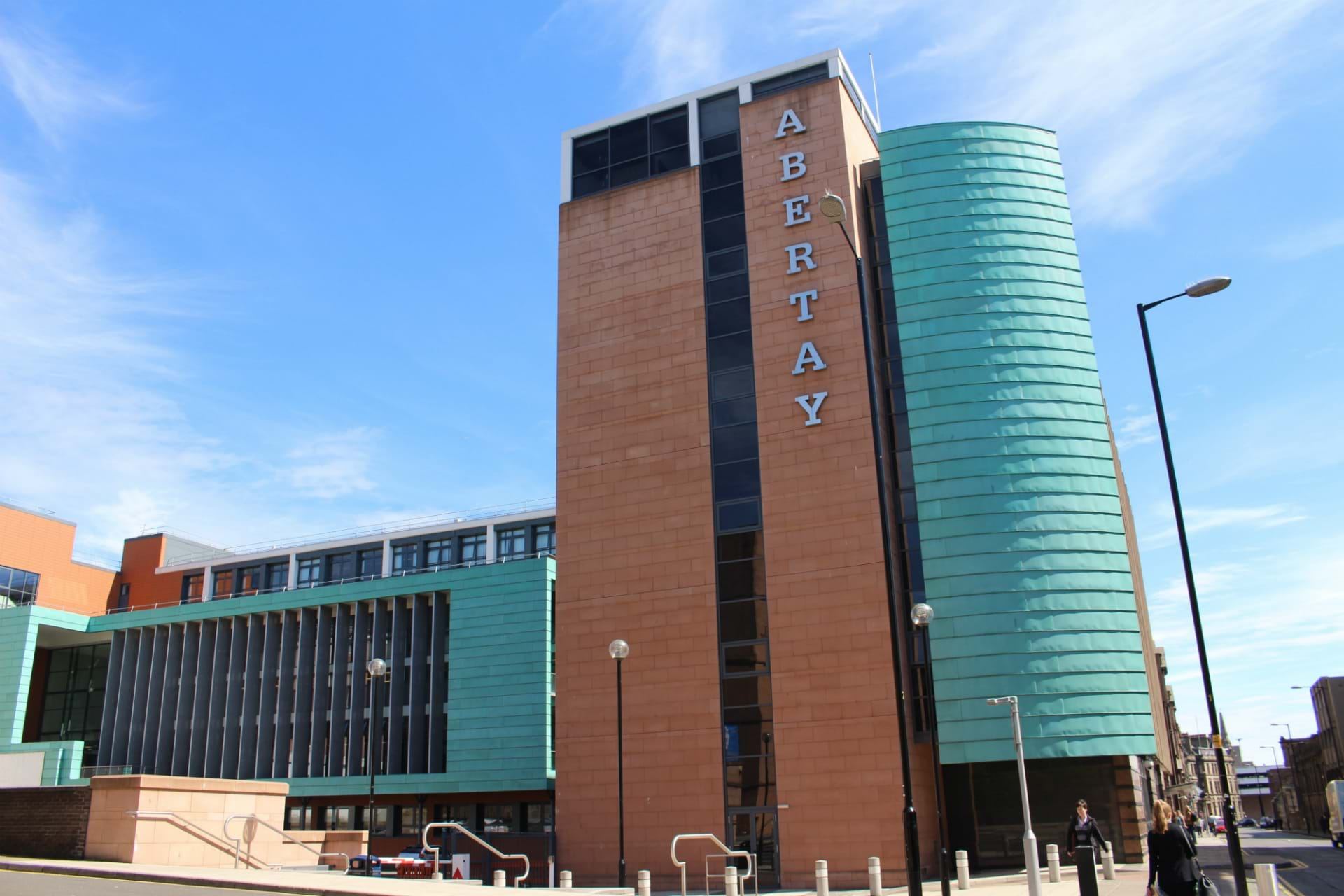
Join Abertay University's vibrant research community
Postgraduate Research students at Abertay work alongside experts from a range of discipline areas, posing new questions and finding creative solutions to the problems facing society. Our research students are central to our research community.
If you'd like more information about our Postgraduate Research degrees, please get in touch:
Related Courses
Water-energy-food nexus (management) msc by research, mphil, phd.
Postgraduate Research Full-Time,Part-Time
This is a potential research degree area, subject to the approval of the...
View course
Anaerobic digestion, biogas & technology MSc by Research, MPhil, PhD
Find a course.
Pause carousel
Play carousel
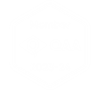
- Bibliography
- More Referencing guides Blog Automated transliteration Relevant bibliographies by topics
- Automated transliteration
- Relevant bibliographies by topics
- Referencing guides
Dissertations / Theses on the topic 'Sustainable urban drainage system'
Create a spot-on reference in apa, mla, chicago, harvard, and other styles.
Consult the top 50 dissertations / theses for your research on the topic 'Sustainable urban drainage system.'
Next to every source in the list of references, there is an 'Add to bibliography' button. Press on it, and we will generate automatically the bibliographic reference to the chosen work in the citation style you need: APA, MLA, Harvard, Chicago, Vancouver, etc.
You can also download the full text of the academic publication as pdf and read online its abstract whenever available in the metadata.
Browse dissertations / theses on a wide variety of disciplines and organise your bibliography correctly.
Swan, Andrew D. "A decision-support system for the design of retrofit sustainable urban drainage systems (SUDS)." Thesis, University of Sheffield, 2003. http://etheses.whiterose.ac.uk/3635/.
Hellberg, Madeleine. "Planning and implementing sustainable urban drainage systems in the built environment." Thesis, Luleå tekniska universitet, Arkitektur och vatten, 2020. http://urn.kb.se/resolve?urn=urn:nbn:se:ltu:diva-82068.
Schlüter, Wolfram. "Behaviour and effectiveness of in-ground sustainable urban drainage systems in Scotland." Thesis, Abertay University, 2005. https://rke.abertay.ac.uk/en/studentTheses/14fd21f3-79a9-4bf7-a082-dd5aecbff0a3.
Tota-Maharaj, Kiran. "Geothermal paving systems for urban runoff treatment and renewable energy efficiency." Thesis, University of Edinburgh, 2010. http://hdl.handle.net/1842/4909.
Macdonald, Kirsteen C. B. "The effectiveness of certain sustainable urban drainage systems in controlling flooding and pollution from urban runoff." Thesis, Abertay University, 2003. https://rke.abertay.ac.uk/en/studentTheses/b6fdd917-aff9-42a2-9b14-089989b57dd2.
Tukiman, Izawati. "Public perception and acceptance of the Sustainable Urban Drainage System (SUDS) in housing schemes in Malaysia." Thesis, University of Sheffield, 2008. http://etheses.whiterose.ac.uk/10327/.
Karlsson, Amanda, and Maria Bergström. "IMPLEMENTERING AV SUSTAINABLE DRAINAGE SYSTEMS I STADSBYGGNADSPROJEKT I JÖNKÖPINGS KOMMUN." Thesis, Tekniska Högskolan, Högskolan i Jönköping, JTH, Byggnadsteknik, 2016. http://urn.kb.se/resolve?urn=urn:nbn:se:hj:diva-31021.
Singh, Rohit. "Integrating the planning of green spaces and sustainable drainage systems." Thesis, Abertay University, 2012. https://rke.abertay.ac.uk/en/studentTheses/0a86d97d-4a27-429a-8dae-6afa03659ca9.
Eckart, Jochen. "Flexible Urban Drainage Systems in New Land-Use Areas." Scholar Commons, 2012. http://scholarcommons.usf.edu/etd/4033.
Robertson, Abby Jane. "Quantifying stormwater pollutants and the efficacy of sustainable drainage systems on the R300 highway, Cape Town." Master's thesis, University of Cape Town, 2017. http://hdl.handle.net/11427/25514.
Lundqvist, Andreas. "The superior option for stormwater management : A case study of Årstafältet." Thesis, KTH, Urbana och regionala studier, 2021. http://urn.kb.se/resolve?urn=urn:nbn:se:kth:diva-298526.
Apostolaki, Stella. "The social dimension of stormwater management practices, including sustainable urban drainage systems and river management options." Thesis, Abertay University, 2007. https://rke.abertay.ac.uk/en/studentTheses/21435036-c7d8-4bd8-b76e-54b26ad63dc2.
Bakhshipour, Amin Ebrahim [Verfasser], and Wolfgang [Akademischer Betreuer] Nowak. "Optimizing hybrid decentralized systems for sustainable urban drainage infrastructures planning / Amin Ebrahim Bakhshipour ; Betreuer: Wolfgang Nowak." Stuttgart : Universitätsbibliothek der Universität Stuttgart, 2021. http://d-nb.info/1234985411/34.
Chunglim, Mak. "Linking sustainable drainage systems (SuDS) together with ecosystem services and disservices : new connections in urban ecology." Thesis, University of Salford, 2015. http://usir.salford.ac.uk/37781/.
Petit, Boix Anna. "Towards sustainable cities through an environmental, economic and eco-efficiency analysis of urban sanitation and drainage systems." Doctoral thesis, Universitat Autònoma de Barcelona, 2017. http://hdl.handle.net/10803/405338.
Harvey, Catherine. "How spatial planning can enable pathways to the implementation of sustainable urban drainage systems in the city bowl, Cape Town." Master's thesis, University of Cape Town, 2018. http://hdl.handle.net/11427/28179.
Torffvit, Felicia. "Dagvattenhantering i hänsyn till kulturmiljön." Thesis, Blekinge Tekniska Högskola, Institutionen för fysisk planering, 2016. http://urn.kb.se/resolve?urn=urn:nbn:se:bth-12911.
Nambinga, Linekela Elias. "Review and gap analysis of Water Sensitive Urban Design (WSUD) in Windhoek, Namibia." Master's thesis, Faculty of Engineering and the Built Environment, 2019. http://hdl.handle.net/11427/30914.
Feder, Marnie Jean. "Towards a rational design for sustainable urban drainage systems : understanding (bio)geochemical mechanisms for enhanced heavy metal immobilization in filters." Thesis, University of Glasgow, 2014. http://theses.gla.ac.uk/5570/.
Silva, Sidnei Pereira da. "Ferramenta de apoio ao manejo de águas pluviais urbanas com base em indicadores de sustentabilidade - SAMSAP." Universidade Federal de São Carlos, 2016. https://repositorio.ufscar.br/handle/ufscar/8575.
Perales, Momparler Carmen Sara. "A regenerative urban stormwater management methodology. The role of SuDS construction and monitoring in the transition of a Mediterranean city." Doctoral thesis, Universitat Politècnica de València, 2015. http://hdl.handle.net/10251/59063.
Smith, Kerry W. S. "Development of a transitioning approach to reduce surface water volumes in combined sewer systems." Thesis, Abertay University, 2016. https://rke.abertay.ac.uk/en/studentTheses/b7c5021f-2efe-421a-b32f-0ac3161fc511.
Schäfer, Benjamin. "Achieving Sustainable Urban Drainage through Multifunctionality." Thesis, KTH, Urbana och regionala studier, 2019. http://urn.kb.se/resolve?urn=urn:nbn:se:kth:diva-257778.
Suteerasan, Sutthi. "Blue-Green Infrastructure on the Move: How Resilience Concepts Travel Between Cities." Thesis, KTH, Urbana och regionala studier, 2021. http://urn.kb.se/resolve?urn=urn:nbn:se:kth:diva-292293.
Mahdian, Adrian. "ECONOMIC AND ENVIRONMENTAL IMPACTS OF STORMWATER MANAGEMENT : Case study: Cost-effectiveness evaluation of Proposition O projects." Thesis, Mälardalens högskola, Akademin för ekonomi, samhälle och teknik, 2020. http://urn.kb.se/resolve?urn=urn:nbn:se:mdh:diva-52945.
Tedoldi, Damien. "Mesure et modélisation de la contamination du sol dans les ouvrages de gestion à la source du ruissellement urbain." Thesis, Paris Est, 2017. http://www.theses.fr/2017PESC1201/document.
Bressy, Adèle. "Flux de micropolluants dans les eaux de ruissellement urbaines : effets de différents modes de gestion à l'amont." Phd thesis, Université Paris-Est, 2010. http://tel.archives-ouvertes.fr/tel-00582379.
Simpson, Murray R. "Sustainable drainage of sports pitches." Thesis, Loughborough University, 2016. https://dspace.lboro.ac.uk/2134/20339.
Aguirre, Herrera Boris Augusto, and Giraldo Melanny Leonor Anchiraico. "Propuesta de utilización de pavimento de concreto permeable para reducir el efecto de precipitaciones intensas en las calles de Alfonso Ugarte y Miguel Grau, ubicadas en el departamento de Tumbes." Bachelor's thesis, Universidad Peruana de Ciencias Aplicadas (UPC), 2021. http://hdl.handle.net/10757/655019.
Oyelola, O. O. "The use of compost and recycled aggregates in the treatment of runoff pollutants in vegetated sustainable drainage devices such as swale." Thesis, Coventry University, 2013. http://curve.coventry.ac.uk/open/items/082ceb3a-f311-4f8e-829f-5120b168724a/1.
Vacková, Michaela. "Urbanistická opatření pro efektivní hospodaření s povrchovou vodou v zastavěných územích." Doctoral thesis, Vysoké učení technické v Brně. Fakulta architektury, 2017. http://www.nusl.cz/ntk/nusl-320340.
Aguirre, B., M. Anchiraico, J. Rodríguez, and F. García. "Sustainable urban pavement for cities affected by El Niño using porous concrete." IOP Publishing Ltd, 2021. http://hdl.handle.net/10757/655955.
Vonck, Kevin J. "Towards more sustainable urban surface drainage a comparative case study of impervious cover policies in Portland, Oregon, and Seattle, Washington /." Access to citation, abstract and download form provided by ProQuest Information and Learning Company; downloadable PDF file, 247 p, 2009. http://proquest.umi.com/pqdweb?did=1833647401&sid=4&Fmt=2&clientId=8331&RQT=309&VName=PQD.
Wang, Fukang. "Real time instruction generator for the Bolton urban drainage control system." Thesis, University of Salford, 1998. http://ethos.bl.uk/OrderDetails.do?uin=uk.bl.ethos.245021.
Alahmari, Ali. "The role of knowledge and institutional challenges to the adoption of sustainable urban drainage in Saudi Arabia : implications for sustainable environmental development." Thesis, Middlesex University, 2018. http://eprints.mdx.ac.uk/25900/.
Fulcher, G. A. "The role of gully pots in determining urban stormwater quality." Thesis, Nottingham Trent University, 1989. http://ethos.bl.uk/OrderDetails.do?uin=uk.bl.ethos.233986.
Camacho, Gema Liliana. "GIS-based tool for assessing hydraulic performance of drainage infrastructure system in El Paso." To access this resource online via ProQuest Dissertations and Theses @ UTEP, 2009. http://0-proquest.umi.com.lib.utep.edu/login?COPT=REJTPTU0YmImSU5UPTAmVkVSPTI=&clientId=2515.
Destro, Cesar Augusto Medeiros. "The urban water use model as a tool to support the evaluation of sustainable drainage measures in Brazilian cities." reponame:Repositório Institucional da UFPR, 2016. http://hdl.handle.net/1884/46507.
Tahir, Osman Mohd. "Urban landscape management in Malaysia : in search of a sustainable management system." Thesis, University of Newcastle Upon Tyne, 2005. http://ethos.bl.uk/OrderDetails.do?uin=uk.bl.ethos.420003.
Napolitano, Lisa. "Analysis and implementation of high resolution precipitation data in urban drainage modelling." Master's thesis, Alma Mater Studiorum - Università di Bologna, 2018.
Altumi, Ahmed Almukhtar. "A decision support system for sustainable urban development of the Tripoli agglomeration, Libya." Thesis, University of Dundee, 2001. http://ethos.bl.uk/OrderDetails.do?uin=uk.bl.ethos.248338.
Muhammad, Imran. "Cities for citizens, not for cars : planning for sustainable urban transport system : case study, Lahore, Pakistan /." Thesis, Hong Kong : University of Hong Kong, 2002. http://sunzi.lib.hku.hk/hkuto/record.jsp?B25247566.
Gökçen, Tankut Serim Erkal. "Influence of urban geometry on public investment cost of urban technical infrastructure:a case study of sewer system in Aydın, Turkey/." [s.l.]: [s.n.], 2005. http://library.iyte.edu.tr/tezlerengelli/doktora/sehirplanlama/T000359.pdf.
Faragher, Tamsin. "Sustainable water governance: An incremental approach towards a decentralised, hybrid water system." Master's thesis, University of Cape Town, 2018. http://hdl.handle.net/11427/29658.
Morales, Pinzón Tito. "Modelling and sustainable management of rainwater harvesting in urban systems." Doctoral thesis, Universitat Autònoma de Barcelona, 2012. http://hdl.handle.net/10803/117610.
Williams, Katie. "The effectiveness of the UK planning system in delivering sustainable development via urban intensification." Thesis, Oxford Brookes University, 1997. http://ethos.bl.uk/OrderDetails.do?uin=uk.bl.ethos.480895.
Dhakras, Bhairavi. "Study of parameters in the development of sustainable transportation system : a case study of Mumbai, India /." See Full Text at OhioLINK ETD Center (Requires Adobe Acrobat Reader for viewing), 2004. http://rave.ohiolink.edu/etdc/view?acc%5Fnum=toledo1091752742.
Karetny, Jane Abigail. "Planning Towards Sustainable Food Systems: An Analysis of U.S. Municipal Food System Plans." The Ohio State University, 2020. http://rave.ohiolink.edu/etdc/view?acc_num=osu1607085379674489.
Hadjimichael, Antonia. "Decision-support for adaptive and sustainable urban wastewater system management in the face of uncertainty." Doctoral thesis, Universitat de Girona, 2016. http://hdl.handle.net/10803/482080.
Greene, Margarita. "From informal settlements to sustainable neighbourhoods : the role of spatial configuration of the urban system." Thesis, University College London (University of London), 2004. http://ethos.bl.uk/OrderDetails.do?uin=uk.bl.ethos.505510.
Thank you for visiting nature.com. You are using a browser version with limited support for CSS. To obtain the best experience, we recommend you use a more up to date browser (or turn off compatibility mode in Internet Explorer). In the meantime, to ensure continued support, we are displaying the site without styles and JavaScript.
- View all journals
- My Account Login
- Explore content
- About the journal
- Publish with us
- Sign up for alerts
- Open access
- Published: 10 September 2021
Towards a model for road runoff infiltration management
- Loïc Maurer 1 , 2 ,
- Julie Zumsteg 1 ,
- Carole Lutz 3 ,
- Marie Pierre Ottermatte 3 ,
- Adrien Wanko 2 ,
- Dimitri Heintz 1 &
- Claire Villette ORCID: orcid.org/0000-0003-2564-5529 1 , 2
npj Clean Water volume 4 , Article number: 44 ( 2021 ) Cite this article
3303 Accesses
2 Citations
2 Altmetric
Metrics details
- Environmental monitoring
- Pollution remediation
- Water resources
In human society, there is a demand for sustainable solutions for water preservation and efficient treatment systems. An important water reservoir is road runoff defined as rainwater leaching from roads, loading micropollutants and infiltrating the soil. We aimed to study this poorly understood feature using large-scale metabolomic analysis coupled with analysis of soil physico-chemical properties and molecular chemical similarity enrichment. A total of 2406 micropollutants were assayed to understand their distribution and assess the trapping abilities of a road runoff infiltration system composed of a sedimentation pond and an infiltration pond. Here, we confirm the essential role of the infiltration pond in preventing environmental contamination and propose a model correlating micropollutant abundance and the soil physico-chemical properties. We demonstrate that sand in infiltration ponds is a key player, helping retain 86% of the micropollutant abundance and propose a model that could be easily applied for road runoff management.
Similar content being viewed by others
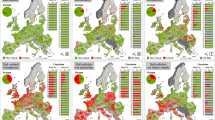
A unifying modelling of multiple land degradation pathways in Europe
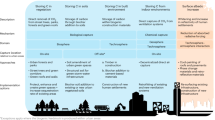
Assessing global urban CO2 removal
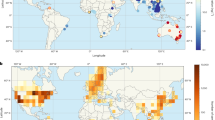
Underestimated burden of per- and polyfluoroalkyl substances in global surface waters and groundwaters
Introduction.
The preservation of water resources is a pressing concern. Road runoff is a non-negligible water input originating from the leaching of roads by rainwater. Little attention has been given to the micropollutants in road runoff and their contribution to environmental pollution. Road runoff can carry fuel, oils, brake fluids, tyre particles, de-icing agents and other pollutants that contain heavy metals, polycyclic aromatic hydrocarbons (PAHs), salts, microplastics, particles, etc. 1 , 2 , 3 , 4 . Phytosanitary products used for road management are also washed off by rain and can reach groundwater as already demonstrated in the case of urban wastewater 5 . The collection and storage of road runoff water prevent the spread of pollutants to surrounding areas and the erosion of banks. Worldwide, underground infiltration 6 , porous pavements, direct infiltration, rain gardens, bioswales and wetlands 7 are used. Sometimes runoff water is collected for reuse 8 , but knowledge about its chemical and molecular content is increasing and these waters have been recognized as being harmful to humans and to the environment 9 and need to be collected and managed as close to their source as possible.
One way to manage the collected water is infiltration, which allows groundwater recharge and reduces peak flows and volumes when the collected water is infiltrated near its collection point 10 , 11 . Infiltration of potentially polluted water raises questions regarding the contamination of soils and groundwater and necessitates an evaluation of the relative risks and benefits 12 . Infiltration ponds play a filtration and adsorption role to prevent the migration of pollutants 13 . Infiltration experiments have been conducted to estimate the fate of pollutants under laboratory conditions, using reconstituted soil columns and artificial runoff water 14 . Few studies were conducted under real conditions 15 as presented here, but the results were promising: these studies showed a reduction in the environmental impact of roads without endangering road integrity, and an increase in aquifer recharge 16 , 17 . The assays were conducted on a shortlist of pollutants, the most cited being metals, chlorides and PAHs 13 , 18 , 19 , 20 . However, some laboratories also worked on larger lists of suspected pollutants or performed research in a non-targeted manner, showing the presence of drugs and pesticides in runoff water or groundwater 21 , 22 , these substances are usually not expected to be present in these matrices and are therefore not searched for.
Knowledge on how soil physico-chemical properties affect micropollutant trapping is scarce, and research is currently focussed on specific pollutant families 23 , 24 . Therefore, the soil properties involved in micropollutant capture during ground infiltration are poorly understood. We measured micropollutant abundance in a road runoff treatment facility and monitored their distribution in sedimentation and infiltration ponds. We measured micropollutant abundance and soil physico-chemical properties at different depths in the infiltration pond from the surface to −165 cm. A chemical enrichment analysis indicated that a sedimentation pond is an indispensable part of every road runoff facility. A correlation analysis between micropollutant abundance and soil physico-chemical properties highlighted the involvement of sand abundance in the retention of micropollutants. Based on these results we propose a holistic model for soil physico-chemical properties affecting micropollutant accumulation in an infiltration pond.
Micropollutant distribution trend in a road runoff facility
The management of road runoff micropollutants is a complex task as a broad diversity of compounds with varied chemical properties must be addressed. The studied system is composed of a sedimentation pond from which water and sediment were analysed, and an infiltration pond from which soil was sampled at different depths (0–10, −80 and −165 cm) (map of the studied site is available in Supplementary Fig. 1 ). A road surface of 3.66 ha is drained to the sedimentation pond of the studied site. It receives 5400 vehicles per day, including ~400 delivery lorries. A total of 94 rain events (at least 1 mm of cumulated rain, the shorter rain events lasting 15 min) were recorded between July 2018 and July 2019, which brought a total of 410.8 mm of rain in 1 year (from 1 to 25 mm per rain event) (Supplementary Dataset 1 ). From water, sediment and soil samples, micropollutants were extracted and identified using mass spectrometry coupled with gas chromatography and liquid chromatography. Alongside these identifications, the physico-chemical properties of the soil from the infiltration pond were investigated. Statistical analyses were performed to investigate the distribution of micropollutants between water, sediments and soil using chemical enrichment, and the correlation between micropollutant abundance and soil physico-chemical properties (Fig. 1a ). From the water, sediment and soil samples, micropollutants were identified and categorized according to their most common usage. Pesticides, drugs, PAHs and toxic industrial chemicals were the most represented categories in terms of the number of micropollutants identified. Figure 1b shows the distribution of these categories among the different samples, as well as the exclusive presence of several micropollutants from a single sample type. These results show the importance of the sedimentation pond, as a higher number of pollutants from all categories were found in water and sediment. Additionally, the highest number of exclusive identifications is depicted in sediment from pesticides (48), drugs (142) and PAHs (4), highlighting the crucial role of this matrix trapping micropollutants and avoiding their spread to the infiltration pond. In the infiltration pond, the soil surface (0–10 cm) retained a high number of micropollutants (184 pesticides, 48 drugs, 16 PAHs and 16 toxic industrial chemicals), reducing the number of compounds identified in the deeper layers of soil (Fig. 1b ). A closer look at the abundance of the compounds confirms the role of the surface layer (0–10 cm), as the micropollutants that were detected in all the soil layers were found to be generally more abundant at the surface of the infiltration pond (Fig. 1c ), with 39–99% of the micropollutants in terms of abundance, trapped in the surface layer (Supplementary Table 1 ). Mostly pesticides and PAHs could be found in all the layers of the infiltration pond soil. PAHs, metals and chlorides, which are common micropollutants encountered in road runoff water, were quantified in the samples (Supplementary Fig. 2 ). PAHs were confirmed to be more abundant in the surface layer of the infiltration pond than in the deeper layers, with 90–99% of the PAHs, in terms of abundance, trapped in the surface layer (Supplementary Table 1 ). Metals and chlorides were not further considered in this study because their concentration was under the French regulation thresholds 25 .
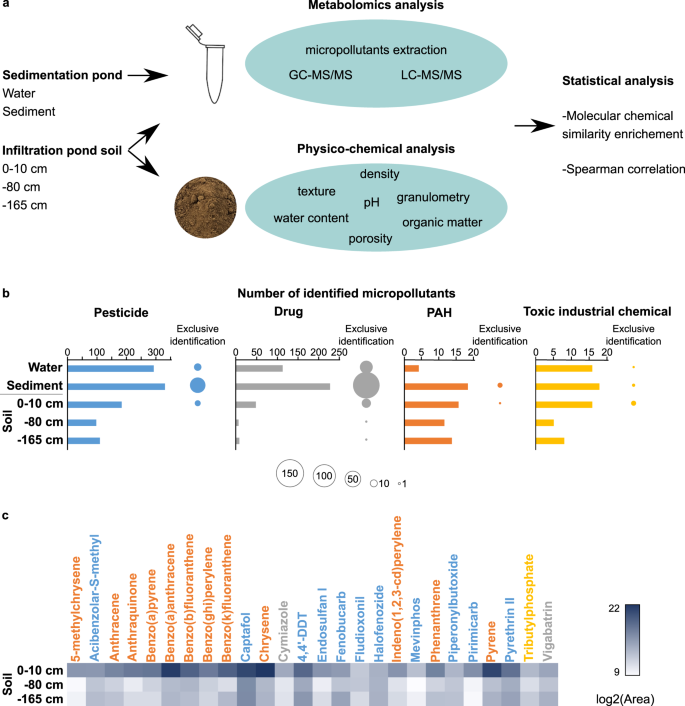
In a first attempt to describe the distribution of micropollutants in the sedimentation pond (water and sediment) and across the soil layers of the infiltration pond (from 0–10 to −165 cm in depth), micropollutants were categorized according to their most common usage: pesticide, drug, PAH and toxic industrial chemical were the prevailing categories identified. a Schematic representation of the study workflow. b Number of micropollutants identified in water, sediment and soil. Exclusive identifications indicate the micropollutants that were identified exclusively in one type of sample. c Distribution of micropollutants in the different layers of the infiltration pond soil presented as log 2 of the peak areas obtained from mass spectrometry analysis.
Chemical enrichment analysis
To better describe the distribution of micropollutants in the studied matrices and to allow comparison with other studies, a chemical enrichment analysis was performed to obtain clusters of micropollutants based on structural similarity and chemical ontology (Fig. 2 ). A pair-wise statistical test was used to highlight increased (red) or decreased (blue) compounds, and clusters containing both increased and decreased compounds are depicted in purple. The size of the circles indicates the number of compounds in the cluster, and only significantly impacted clusters of a minimum of three compounds are shown on the graph. The position of the clusters on the x -axis depends on their partition coefficient, expressed as the median XlogP value (hydrophilic compounds on the left and hydrophobic compounds on the right).
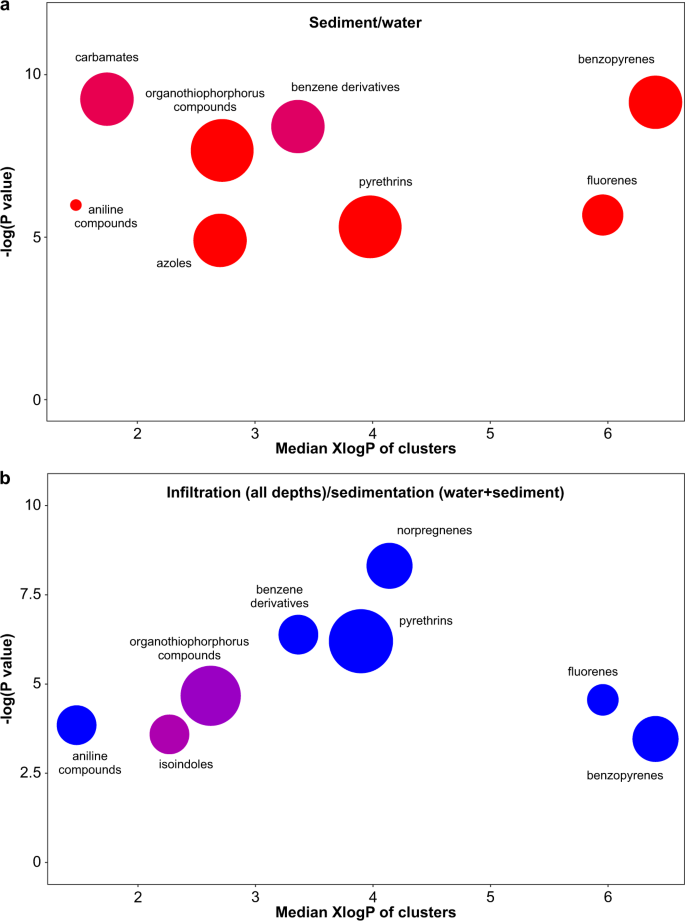
Significantly impacted metabolite clusters ( P < 0.05) are depicted in red (increased) and blue (decreased). The most significantly altered clusters are shown on the top of the y -axis; clusters are distributed on the x -axis based on their partition coefficient (hydrophilic compounds on the left and hydrophobic compounds on the right). The size of the circles indicates the number of compounds in each cluster. a Chemical enrichment between sediment and water and b chemical enrichment analysis between infiltration (all depth) and sedimentation (water + sediment). Chemical enrichment statistics were calculated by the Kolmogorov–Smirnov test.
The first comparison was made between sediment and water (Fig. 2a ) to understand the behaviour of micropollutants in the sedimentation pond and their distribution among the liquid and solid matrices. The distribution of the clusters on the x -axis depicts a rather high variability in terms of hydrophobicity, ranging from very hydrophilic aniline compounds to very hydrophobic benzene derivatives and fluorenes (PAHs). Nevertheless, all the clusters are coloured red, which indicates that all the compound families are increased in the sediment samples compared to the water samples. Micropollutants trapped in sediment do not seem to be released to the infiltration pond, as demonstrated by the chemical enrichment analysis between the infiltration pond (all layers) and the sedimentation pond (water and sediment) (Fig. 2b ). All but two clusters were decreased in the infiltration pond, and almost the same clusters appeared in this analysis compared to the sediment/water comparison. Organothiophosphorus compounds and isoindoles are depicted in purple, which means that only part of the compounds from this family was increased, while others were decreased (details are available in Supplementary Dataset 2 ). Chemical enrichment analysis revealed six clusters that were always found to be significantly impacted, regardless of the pairwise comparison made: aniline compounds, organothiophosphorus compounds, benzene derivatives, pyrethrins, benzopyrenes and fluorenes. The analysis also confirms the crucial role of the sedimentation pond in trapping micropollutants (in terms of not only in the number of micropollutants but also in abundance), which are mostly retained by sediment, regardless of their hydrophilicity, while logP (also known as log Kow) is often said to be one of the key indicators of micropollutant distribution in the environment 26 .
Correlation of soil properties and micropollutant abundance
To understand the fate of micropollutants in the soil during infiltration, three clusters with distinct hydrophobicity were chosen (Fig. 2 ): aniline compounds (median XlogP < 2), pyrethrins (median XlogP ≈ 4) and benzopyrenes, which were grouped with fluorenes (median XlogP > 6), as these two clusters are described as PAHs in the literature even though the chemical ontology separates them. These three clusters were used to investigate the correlations between compound abundance and soil physico-chemical properties (Fig. 3 ). Correlation coefficients were interpreted as negligible to highly positive/negative as described in the literature 27 .
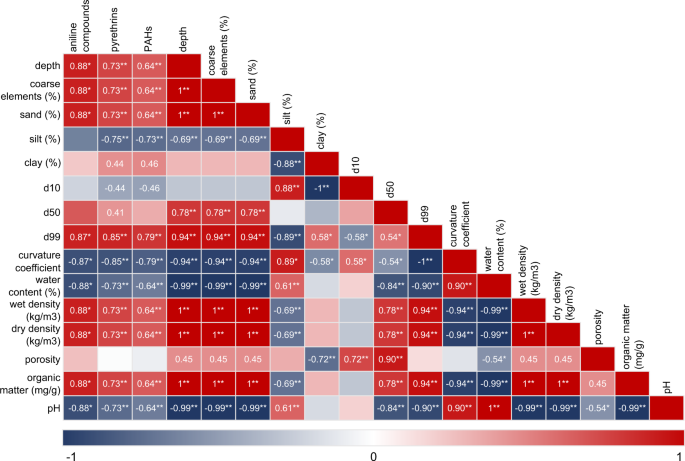
Correlation coefficients were calculated using Spearman’s correlation; positive coefficients (red) indicate a positive relationship between the variables, and negative coefficients (blue) indicate a negative relationship. Values near zero (white) indicate no relationship between the variables. Correlation coefficients are indicated only if P < 0.05; asterisks indicate P < 0.01 (*) and P < 0.001 (**). N = 9 (aniline compounds), 24 (pyrethrins), 27 (PAHs). d10, 1.26–1.38 µm particles; d50, 20.7–22.73 µm particles; d99, 101.1–161.2 µm particles.
The first piece of striking evidence is that the positive or negative trends were the same for all micropollutant families, regardless of the median XlogP value. A gradient was observed, with higher absolute values of the correlation coefficients for the compounds with lower median XlogP values. The abundance of aniline compounds was positively correlated with depth, percentage of coarse elements and sand, soil density and organic matter content, with a high correlation coefficient of 0.88 ( P < 0.01) for all correlations. This indicates that the abundance of the compounds increased as these soil parameters increased. The abundance of aniline compounds was negatively correlated with water content and soil pH with a high correlation coefficient of −0.88 ( P < 0.01), which indicates that the abundance of the compounds decreased when water content and pH increased. The presence of sand and clay seemed to help in PAH capture, as moderate positive correlations were observed (0.64 ( P < 0.001) and 0.46 ( P < 0.05) respectively) while the percentage of silt was highly negatively correlated with PAH abundance (−0.73, P < 0.001). Pyrethrins showed the same trends as aniline compounds and PAHs, with correlation coefficients (absolute values) between those of the two other compound families.
The curvature coefficient obtained for the different soil layers was between 4.05 and 4.44; therefore, the soil in the infiltration pond was not well graded. The defined particle sizes were 1.26–1.38 µm (d10), 20.7–22.73 µm (d50) and 101.1–161.2 µm (d99) (Supplementary Table 2 ). The largest particles of the soil (>100 µm) were the best suited to retain micropollutants with a high positive correlation between micropollutant abundance and the d99 value (0.87, P < 0.05; 0.85, P < 0.01; 0.79, P < 0.001). Indeed, smaller particles showed a low negative correlation with pyrethrin and PAH abundance (−0.44 and −0.46, respectively, P < 0.05) and the high to moderate positive correlations between micropollutant abundance and the percentage of coarse elements confirm this hypothesis (0.88, P < 0.01; 0.73, P < 0.001 and 0.64, P < 0.001). The water content (19–21%) and pH value (6.7–7.1) did not vary much between the layers of the soil and did not seem to help in micropollutant trapping in the surface layer of the infiltration pond. The abundance of micropollutants increased as the water content decreased (−0.8, P < 0.01; −0.37, P < 0.001; −0.64, P < 0.001), but in an infiltration pond, it is not possible to reduce the amount of water since the primary function of the pond is infiltration. Regarding pH, the correlation coefficients were the same as those for water content, indicating that micropollutant abundance increased when pH decreased, which can be managed if necessary. Porosity was not correlated with the abundance of any of the compound families presented here.
The content of organic matter was high to moderately correlated with micropollutant abundance (0.88, P < 0.01; 0.73, P < 0.001, 0.64, P < 0.001). The organic matter was highest in the surface layer (6 mg/g) and decreased with depth (−80 cm, 5.2 mg/g; −165 cm, 4.7 mg/g). As the soil surface layer also harbours living organisms and plant root systems, the ratio of plant root systems and soil was investigated at four distinct sampling points situated 30 cm around the coring site (Table 1 ). The presence of small organisms and microorganisms could not be studied due to the lack of technical means.
Roots in the top 10 cm of the infiltration pond represented between 10.9 and 35.5% of the total soil dry weight (Table 1 ), indicating that micropollutants identified in the surface layer of the infiltration pond might interact with not only mineral matter but also living organisms, including plant roots that can represent up to 1/3 of the soil dry weight. The plant diversity at the studied site was important, and many spontaneous plants settled at this location by themselves. The water content was comparable between roots and soil samples for each sampling area (Table 1 ), helping micropollutant exchange through the water flux between soil particles and plant roots.
The distribution of a complex mixture of road runoff micropollutants was investigated in a treatment facility composed of a sedimentation pond, followed by an infiltration pond. Of the 2406 micropollutants investigated, 700 were identified in the different matrices studied. The critical role of the sedimentation pond was demonstrated here, not only in terms of the number of micropollutants retained (610 in total, Fig. 1 ) but also with regard to the abundance of micropollutants (Fig. 2 ) with a mean trapping of 79% of the abundance of micropollutants, reaching 91–98% for PAHs (Supplementary Table 1 ). The identified micropollutants were distributed mainly in the sediment part of the pond, with only a few identified compounds specific to the water part (33 drugs, 11 pesticides and 1 toxic industrial chemical). One could have expected hydrophilic compounds to stay in solution in the water matrix and hydrophobic compounds to be sequestered by suspended solids and to settle and form sediment 28 , 29 . However, this is a very favourable result, as it shows that micropollutants were trapped in the sedimentation pond and that release to the infiltration pond and to the environment was avoided. The presence of the sedimentation pond prevents the spread of micropollutants, thereby protecting soil and groundwater from contamination, as previously described in the literature 30 , 31 , 32 .
Moreover, the surface layer of the infiltration pond showed high efficiency in retaining micropollutants from infiltrating into the deeper layers of the soil. The observation that road runoff pollutants are sequestered in the surface layers of soils has already been made in the previous studies 13 , 33 , 34 . The number of micropollutants trapped in the surface layer is important (269 micropollutants, Fig. 1b ), and the abundance of micropollutants detected in the surface layer is much more important than in the other layers, with a mean value of 86% of the abundance of micropollutants trapped at the surface (Supplementary Table 1 , Fig. 1c and Supplementary Fig. 2 ). The correlation analysis with soil properties provides some clues regarding how to maintain and increase the natural abilities of the infiltration soil to retain micropollutants.
A previous study that focussed on per- and polyfluoroalkyl substances already showed that multiple soil properties are involved in pollutant retention 23 . Other studies also present interesting results regarding soil physico-chemical parameters as pH or organic matter content affecting the soil–solution partitioning of metals 26 , 35 , 36 . Here, a correlation analysis was performed using three chemical families of micropollutants with varied median XlogP values, to identify general observations suitable for most of the micropollutants encountered in road runoff facilities. The positive and negative correlation trends were the same for all the considered families, and a gradient was observed in the correlation coefficients, with higher coefficients (absolute value) for compounds with lower median XlogP values. The proposed results are applicable to pollutants with median XlogP values ranging from <2 to >6, as presented in this study. Five soil properties were identified as being negatively correlated with micropollutant abundance, while seven properties were identified as being positively correlated (Fig. 4a ). The analysis shows that a higher proportion of sand with a large particle size was better suited for the retention of micropollutants with different median XlogP values in the surface layer of the infiltration pond. The presence of clay might aid the trapping of micropollutants with higher median XlogP values. Silt and particles with a size <20 µm should be avoided. The studied soil properties are known to affect soil permeability, which might impact the retention time and therefore the adsorption capacity of the infiltration pond soil towards micropollutants. The soil pH value was negatively correlated with micropollutant abundance; therefore, decreasing the pH of the infiltration pond soil could be a suitable management practice for use in infiltration ponds. The correlation between pH and the proportion of sand was very highly negative (−0.99, P < 0.001), indicating that increasing the proportion of sand would lower the pH, and therefore help in micropollutant trapping as the micropollutant abundance increases with increasing amounts of sand and decreasing pH. The organic matter content was very highly correlated with the proportion of sand in the pond. Once again, increasing the proportion of sand would increase the organic matter content and help in micropollutant retentions. Moreover, better retention of PAHs in soil fractions containing organic matter has been previously reported 37 . A management strategy is proposed, wherein the central element is the proportion of sand in the soil (Fig. 4b ). Sand is mainly composed of silica, which is used in chromatography to adsorb molecules according to their polarity. Therefore, we hypothesize that sand particles with a large size (>100 µm) offer a large contact surface and might be well suited to help the adsorption of micropollutants, to trap them and avoid infiltration to the deeper layers of the soil. A moderate efficiency of sand in the removal of micropollutants has already been described 38 . In addition to this adsorption capacity, sand is a good host for bacteria, which form a biofilm able to accumulate micropollutants. It has been demonstrated that the presence of a biofilm in a sand filter increases its micropollutant removal efficiency 39 . Therefore, the role of sand in micropollutant removal would be due in part to adsorption, but also to accumulation in the biofilm.
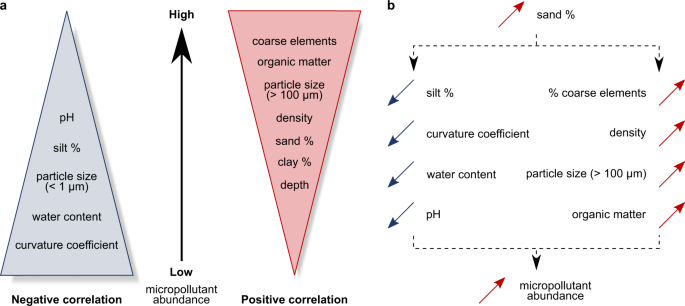
a Soil properties could be divided into those that are negatively (blue) and positively (red) correlated with micropollutant abundance. b A strategy is proposed to increase micropollutant trapping in the soil of an infiltration pond.
One should also keep in mind that biodiversity in soils is known to have a role in micropollutant trapping and biodegradation. The Food and Agriculture Organization stated that “One teaspoon of soil contains more living organisms than there are people in the world” 40 . One gram of soil can contain up to 1 billion bacterial cells, 200 m of fungal hyphae and several thousand different taxa of macroorganisms, and the total biomass below ground would equal or potentially exceed that above ground 40 . Therefore, the role of soil microorganisms in trapping and transforming micropollutants should be considered. These organisms range from 20 nm to 20–3 cm in body size and can be divided into four size classes: microbes (20 nm–10 µm), mesofauna (0.1–2 mm), macrofauna (2–20 mm) and megafauna (>20 mm) 40 . Soil microorganisms participate in soil structure, decomposition of organic matter, water retention, soil aeration and movement of soil particles through different layers. For example, bacteria have already been described to be the most active in the top 10 cm of soil in the context of constructed wetlands 41 . The size of soil particles and the size of organisms are closely related: larger organisms (from vertebrates to nematodes) are found in sandy soils; fungi, protozoa and some algae are found in silt; and other bacteria, archaea and viruses are found in clay 42 . Plants can establish their roots in sandy to silty soils and interact closely with soil organisms. They perform phytoextraction in their aerial parts and phytostabilization in their underground root system through absorption and accumulation, adsorption onto roots or precipitation within the root zone, which decreases the bioavailability of micropollutants in the soil and reduces ecotoxicity 43 . Plant diversity is a key player in micropollutant management, as each plant manages a distinct pool of micropollutants, with some of them being specific to certain plants 44 . Spontaneous plants are well suited for micropollutant management, as they naturally adapt to the constraints of the environment, settle only if they can survive in the presence of micropollutants and do not need any care. Preserving the natural biodiversity in facilities is also a way to avoid the spread of micropollutants.
From the results obtained in this study, a model is proposed for soil physico-chemical parameters that affect micropollutant abundance in a road runoff infiltration pond, regardless of their median XlogP value. These results are applicable for micropollutants under real conditions in a road runoff treatment facility composed of a sedimentation pond and an infiltration pond. The curvature coefficient, water content, small soil particles (<1 µm), silt proportion and pH are soil physico-chemical parameters that are negatively correlated with micropollutant abundance. This means that these parameters decrease when the abundance of micropollutants increases. The presence of coarse elements, organic matter, large soil particles (>100 µm), density and the proportions of sand and clay are positively correlated with micropollutant abundance, which means that these parameters increase when micropollutant abundance increases.
To help in micropollutant trapping in an infiltration pond and avoid leaching to groundwater, a simple solution seems to be to increase the proportion of sand in the infiltration soil. Indeed, the proportion of sand is positively correlated with the presence of coarse elements and large particles (d99), density and organic matter content, properties that are positively correlated with micropollutant abundance. Moreover, the proportion of sand is negatively correlated with the proportion of silt, water content, curvature coefficient and pH, which are negatively correlated with micropollutant abundance. Therefore, increasing the proportion of sand would decrease negatively correlated parameters and help in micropollutant trapping. In addition to allowing and preserving the settlement of the natural biodiversity in the facilities, increasing the amount of sand in the infiltration pond is an easy solution that seems to interact well with all the studied soil physico-chemical parameters according to the correlation analysis. This solution is inexpensive and demands reduced primary inputs; moreover, the presence of small-scale vegetated sedimentation and infiltration ponds along roads is socially desirable and helps manage road runoff pollution at its source to prevent environmental contamination.
Study site and sampling
The study site is situated in Wolfisheim, Alsace (France), along department road RD45 (48°35′38.353″N, 7°39′19.652″E) and was established in 2013. It is composed of a sedimentation pond collecting road runoff from 3.66 ha of road, followed by an infiltration pond to release water to the environment (a detailed view of the study site is provided in Supplementary Fig. 1 ). The sedimentation pond is lined and made of concrete to avoid the infiltration of runoff water at this step. Suspended solids from runoff water, which settled on the concrete floor of the sedimentation pond, were collected and referred to as “sediment” in all the manuscripts. Water and sediment were sampled from the entrance of the sedimentation pond. Soil from the infiltration pond and from the bank (used as a control) was sampled at the surface (0–10 cm), at −80 cm and at −165 cm using a manual auger. Three samples of each matrix were collected as replicates (individual samples that are considered representative of the studied matrix). All samples were stored in glass bottles and immediately placed at 4 °C in the dark for transport. Upon arrival at the laboratory, weighing of the sediment/soil and filtering of the water using a coffee filter were performed in a cold room (4 °C). Pollutants were extracted from fresh sediment and soil samples in the dark and without drying (see ‘Correlation of soil properties and micropollutant abundance’). A weather station (Adcon Telemetry) was implemented on the site to record rain events every 15 min (Supplementary Dataset 1 ).
Four samples of surface soil (0–10 cm) were collected at 30 cm from the drilling point in four opposite directions to analyse the proportion of roots and soil in the samples. Roots were manually sorted from soil particles using surgical pliers. Samples were dried in an oven at 105 °C for 4 h before weighing the dry weight.
Solvents (acetonitrile, methanol and isopropanol) were purchased from Fisher Chemicals (New Hampshire, USA). Acetic and formic acids were purchased from Sigma-Aldrich (Missouri, USA), ammonium formate was purchased from Fluka Analytical (Missouri, USA) and NaOH was purchased from Agilent Technologies (California, USA). Deionized water was obtained from a Direct-Q UV station (Millipore, Massachusetts, USA). Pure standards of PAHs used for quantification were purchased as a mix from Restek (Lisses, France), ref. 31455. Analytical performance quality checks were performed with a mix of pesticides obtained from Restek (ref. 32563 for gas chromatography and refs. 31972 and 31978 for liquid chromatography).
Soil physico-chemical property measurements
All the soil layers were wet sieved at 2 mm and stored wet at 4 °C before soil characterization. Physico-chemical parameters were measured following standard analytical procedures (NF/ISO) on homogenized sediment samples, dried at 105 °C and sieved to 2 mm. Soil water content was measured by the mass difference after drying to constant mass (ISO 11465). The particle size distribution was measured by laser diffraction methods in water mode (LS230, Beckmann Coulter; ISO 13320). The results from the distribution were used to cluster the soil using three texture class levels following the World Reference Base 45 of soil: clay <2 µm, silt from 2 to 50 µm and sand from 50 to 2000 µm. Then, solid–liquid extraction was used to remove the following phases: (1) organic matter was extracted by a 1:3 (v/v) solution of H 2 O 2 at 60 °C, (2) cationic flocculants were extracted using KCl (1:50 (v/v)) or HCl (1:20 (v/v)) depending on the carbonate content of the sample and (3) desegregation of particles was performed by adding a 1:1 (v/v) solution of sodium hexametaphosphate 0.55%. Bulk density and porosity were obtained by following the French standard NF P94-410-3. The porosity measurement was performed using a water pycnometer. Organic matter content was determined by measuring the weight loss at 550 °C for 24 h (in-house method). The pH value was measured by following the standard NF EN 15933.
Metal and chloride analysis
The samples were analysed by EUROFINS (Saverne, France) for the quantification of aluminium, cadmium, copper, nickel, lead and mercury. Mercury was quantified using cold vapour atomic fluorescence spectroscopy following the French standard NF EN 13346-NF ISO 16772. Other metals were quantified using inductively coupled plasma-atomic emission spectroscopy following the French standard NF EN ISO 11885-NF EN 13346. Chlorides were quantified using potentiometry following the French standard NF EN ISO 10304.
Micropollutant extraction
The extraction methods for micropollutants from water and soil were developed in the laboratory using internal standards in representative samples, as already published by Villette et al. 46 . Briefly, pollutants were extracted from 10 g of soil by performing a double extraction. The first overnight extraction was performed using 40 mL of acetonitrile:H 2 O (90:10) and 1% acetic acid at 4 °C under shaking with a magnetic stirrer. The samples were centrifuged for 15 min at 5827 × g to collect the supernatant. A second extraction was applied to the pellet using 20 mL of isopropanol:acetonitrile (90:10) for 15 min at 4 °C under shaking. After a second centrifugation, the supernatants were pooled and freeze-dried. Samples were solubilized in 1 mL of acetonitrile:isopropanol:H 2 O (50:45:5) and diluted 10 times in H 2 O for liquid chromatography-high-resolution mass spectrometry (LC-HRMS) analysis or solubilized in 1 mL of ethyl acetate and diluted 2 times for gas chromatography–tandem mass spectrometry (GC-MS/MS) analysis.
Fifty millilitres of groundwater samples were freeze-dried under vacuum and recovered in 1 mL of MeOH:H 2 O (90:10) and diluted 10 times in water for LC-HRMS analysis or recovered in 1 mL ethyl acetate and diluted 2 times for GC-MS/MS analysis.
LC-quadrupole-time-of flight-HRMS analysis
Samples were analysed by LC coupled with HRMS using a DioneX Ultimate 3000 (Thermo, Massachusetts, USA) coupled to a Q-TOF Impact II (Bruker, Bremen, Germany) using the TargetScreener method (Bruker), which allows targeted identification of micropollutants using a database containing 2072 pollutants (848 pesticides and 1224 toxicants), their retention time, their exact mass and the exact mass of their fragments in broad-band collision-induced dissociation (bbCID) fragmentation mode. Samples were analysed on a C18 column (Acclaim TM RSLC 120 C18, 2.2 µm, 120 A, 2.1 × 100 mm 2 , Dionex bonded silica products) equipped with an Acquity UPLC® BEH C18 pre-column (1.7 µm, 2.1 × 5 mm 2 ) using a gradient of solvent A (H 2 O:MeOH 90:10, 0.01% formic acid, 314 mg L −1 ammonium formate) and solvent B (MeOH, 0.01% formic acid, 314 mg L −1 ammonium formate) as described in detail by Villette et al. 46 . The spectrometer was operated in positive ion mode using bbCID fragmentation mode in a mass range from 30 to 1000 Da, with a spectra rate of 2 Hz. Analytical quality checks were performed using a mix of pesticides to assess the retention times (refs. 31972 and 31978, Restek).
GC-MS/MS analysis
GC-MS/MS analysis was performed using GC coupled to a triple quadrupole detector (SCION 436-MS, Bruker). Samples were carried through a Rxi ® -5Sil MS column (Restek, 30 m, 0.25 mm ID, 0.25 µm) with a constant flow (1 mL min −1 ) of helium gas (Alphagaz 2, Air Liquide). The injector was operated at 280 °C with a pressure pulse at 30 psi, the oven was set at 70 °C for 0.7 min and the temperature was increased to 180 °C at 30 °C/min, and then to 300 °C at 10 °C/min, with a stabilization time of 2 min. The spectrometer was operated in multiple reaction monitoring (MRM) scan type with an electron energy of 70 eV to search for 328 micropollutants (pesticides, PAHs and toxicants). At least two daughter ions were recorded for each analyte, and a retention window of 5 min was used. For quantification, a mix of PAH analytical standards (EPA 610 B, ref. 31455, Restek) was used and injected using the same method, and then standard curves were established manually. Analytical quality checks were performed using a mix of pesticides to assess the retention times (ref 32563, Restek).
MS data analysis
Micropollutants were identified to level 1 of the Schymanski classification 47 based on their retention time, exact mass of the parent ion and fragment ions when available using LC and GC. For LC-HRMS data analysis, the signal-to-noise ratio was set to 3, the retention time window was set to 0.25 min and the exact mass variation was set to 3 p.p.m. in the TASQ software (Bruker). A list of 2072 micropollutants was investigated, and the identification was confirmed based on the retention time, exact mass, isotopic pattern of the parent ion and at least one daughter ion obtained from the bbCID collision mode used on the spectrometer; identification was performed via interrogation of pesticide and toxic chemical databases. For GC-MS/MS data analysis, the signal-to-noise ratio was set to 3, the retention time window was set to 0.25 min and the mass variation was set to 0.5 Da in MS Data Review software 8.2 (Bruker). The presence of at least two daughter ions obtained from the MRM mode on the spectrometer confirmed the identification results from the list of 328 micropollutants investigated.
Labelled internal standards were used to assess the repeatability of the extraction process and to determine the limits of detection and quantification 46 , and the coefficients of variation obtained were in accordance with already published literature 48 .
Statistical analysis
Statistical analyses of the whole dataset were performed using the area of the peaks as the unit of reference. The dataset obtained from LC-HRMS and GC-MS/MS experiments (see Section 2.7) and containing the names of the compounds and the areas of the peaks in the different soil layers was imported into Metaboscape 4.0 (Bruker) as a csv file for statistical analysis. The thresholds used to indicate a significant difference were P ≤ 0.05 and fold change ≥2 or ≤−2. Due to the small number of samples (three replicates for each condition), the nonparametric Wilcoxon’s rank-sum test was used.
Chemical enrichment analysis was performed on the pollutants identified using the online version of the ChemRICH tool 49 . To generate the dataset for ChemRICH interrogation, chemical identifiers (PubChem ID, InChIKey and SMILE) were manually recovered from the names of the compounds using the PubChem Identifier Exchange Service ( https://pubchem.ncbi.nlm.nih.gov/idexchange/idexchange.cgi ). These identifiers are necessary for the tool to estimate the structural similarity between the compounds based on chemical ontologies. The statistical information was then used to propose a statistical enrichment approach and highlight the compounds that were up- or downregulated under a specific condition. The whole dataset (significantly differential and non-differential values) was submitted to ChemRICH, with the fold change converted to the average ratio. The ChemRICH thresholds were P ≤ 0.05 and fold change ≥2 or ≤−2 to indicate whether a compound was significantly up- or downregulated under a specific condition and to obtain the chemical enrichment analysis results.
The correlation coefficients between micropollutant abundance and soil physico-chemical properties were calculated using Spearman’s correlation due to the small number of observations and the presence of continuous and ordinal variables 27 . The correlation matrix was created from the obtained coefficients and is presented with a gradient of blue (negative correlation) to red (positive correlation). The coefficients are indicated in the matrix only if P < 0.05 for the corresponding correlation. Correlation coefficients were considered very high (0.9–1.0) to negligible (0.0–0.3) according to a previously proposed ref. 27 .
For quantification data, statistical analysis was performed in R3.6.1 to search for significant differences in metal, chloride and PAH concentrations between the bank and the pond.
Data availability
The datasets generated and analysed during the current study are available from the corresponding author on reasonable request.
Leroy, M. C. et al. Assessment of PAH dissipation processes in large-scale outdoor mesocosms simulating vegetated road-side swales. Sci. Total Environ. 520 , 146–153 (2015).
Article CAS Google Scholar
Helmreich, B., Hilliges, R., Schriewer, A. & Horn, H. Runoff pollutants of a highly trafficked urban road - correlation analysis and seasonal influences. Chemosphere 80 , 991–997 (2010).
Wagner, S. et al. Tire wear particles in the aquatic environment - A review on generation, analysis, occurrence, fate and effects. Water Res. 139 , 83–100 (2018).
Pramanik, B. K., Roychand, R., Monira, S., Bhuiyan, M. & Jegatheesan, V. Fate of road-dust associated microplastics and per- and polyfluorinated substances in stormwater. Process Saf. Environ. Prot. 144 , 236–241 (2020).
Hensen, B. et al. Entry of biocides and their transformation products into groundwater via urban stormwater infiltration systems. Water Res. 144 , 413–423 (2018).
Mrowiec, M. Road runoff management using improved infiltration ponds. Transp. Res. Procedia 14 , 2659–2667 (2016).
Article Google Scholar
Goh, X., Radhakrishnan, M., Zevenbergen, C. & Pathirana, A. Effectiveness of Runoff control legislation and active, beautiful, clean (ABC) waters design features in Singapore. Water 9 , 627 (2017).
Liu, A., Liu, L., Li, D. & Guan, Y. Characterizing heavy metal build-up on urban road surfaces: Implication for stormwater reuse. Sci. Total Environ. 515–516 , 20–29 (2015).
Chen, C., Guo, W. & Ngo, H. H. Pesticides in stormwater runoff—a mini review. Front. Environ Sci. Eng. 13 , 72 (2019).
Leroy, M. C. et al. Performance of vegetated swales for improving road runoff quality in a moderate traffic urban area. Sci. Total Environ. 566–567 , 113–121 (2016).
Weiss, P. T., LeFevre, G. & Gulliver, J. S. Contamination of Soil and Groundwater due to Stormwater Infiltration Practices . Saint Anthony Falls Laboratory Project Report No. 38 (Saint Anthony Falls Laboratory, 2008).
Cederkvist, K., Jensen, M. B. & Holm, P. E. Method for assessment of stormwater treatment facilities – synthetic road runoff addition including micro-pollutants and tracer. J. Environ. Manag. 198 , 107–117 (2017).
Tedoldi, D., Chebbo, G., Pierlot, D., Kovacs, Y. & Gromaire, M. C. Impact of runoff infiltration on contaminant accumulation and transport in the soil/filter media of Sustainable Urban Drainage Systems: a literature review. Sci. Total Environ. 569–570 , 904–926 (2016).
Murakami, M. et al. Multiple evaluations of the removal of pollutants in road runoff by soil infiltration. Water Res. 42 , 2745–2755 (2008).
Flanagan, K. et al. Retention and transport processes of particulate and dissolved micropollutants in stormwater biofilters treating road runoff. Sci. Total Environ. 656 , 1178–1190 (2019).
Piguet, P., Parriaux, A. & Bensimon, M. The diffuse infiltration of road runoff: An environmental improvement. Sci. Total Environ. 397 , 13–23 (2008).
Scholz, M. & Kazemi Yazdi, S. Treatment of road runoff by a combined storm water treatment, detention and infiltration system. Water Air Soil Pollut. 198 , 55–64 (2009).
Huber, M. & Helmreich, B. Stormwater management: calculation of traffic area runoff loads and traffic related emissions. Water 8 , 294 (2016).
Krein, A. & Schorer, M. Road runoff pollution by polycyclic aromatic hydrocarbons and its contribution to river sediments. Water Res. 34 , 4110–4115 (2000).
Murakami, M., Nakajima, F. & Furumai, H. Modelling of runoff behaviour of particle-bound polycyclic aromatic hydrocarbons (PAHs) from roads and roofs. Water Res. 38 , 4475–4483 (2004).
Pinasseau, L. et al. Use of passive sampling and high resolution mass spectrometry using a suspect screening approach to characterise emerging pollutants in contaminated groundwater and runoff. Sci. Total Environ. 672 , 253–263 (2019).
Bergé, A. et al. Non-target strategies by HRMS to evaluate fluidized micro-grain activated carbon as a tertiary treatment of wastewater. Chemosphere 213 , 587–595 (2018).
Nguyen, T. M. H. et al. Influences of chemical properties, soil properties, and solution ph on soil-water partitioning coefficients of per- and polyfluoroalkyl substances (PFASs). Environ. Sci. Technol. 54 , 15883–15892 (2020).
Batjes, N. H. Methodological Framework for Assessment and Mapping of the Vulnerability of Soils to Diffuse Pollution at a Continental Level (SOVEUR Project) (ISRIC—World Soil Information, 1997).
Arrêté du 8 janvier 1998 fixant les prescriptions techniques applicables aux épandages de boues sur les sols agricoles pris en application du décret no. 97-1133 du 8 décembre 1997 relatif à l’épandage des boues issues du traitement des eaux usées. J. Off. 16 , https://www.legifrance.gouv.fr/loda/id/JORFTEXT000000570287/ (1998).
Sauvé, S., Hendershot, W. & Allen, H. E. Solid-solution partitioning of metals in contaminated soils: dependence on pH, total metal burden and organic matter. Environ. Sci. Technol. 34 , 1125–1131 (2000).
Yadav, S. Correlation analysis in biological studies. J. Pract. Cardiovasc. Sci. 4 , 116 (2018).
Cottin, N. & Merlin, G. Removal of PAHs from laboratory columns simulating the humus upper layer of vertical flow constructed wetlands. Chemosphere 73 , 711–716 (2008).
Ren, X. et al. Sorption, transport and biodegradation – an insight into bioavailability of persistent organic pollutants in soil. Sci. Total Environ. 610–611 , 1154–1163 (2018).
Wiest, L. et al. Priority substances in accumulated sediments in a stormwater detention basin from an industrial area. Environ. Pollut. 243 , 1669–1678 (2018).
Hares, R. J. & Ward, N. I. Sediment accumulation in newly constructed vegetative treatment facilities along a new major road. Sci. Total Environ. 334–335 , 473–479 (2004).
Strömvall, A., Norin, M. & Pettersson, T. J. R. Organic contaminants in urban sediments and vertical leaching in road ditches. In The Eighth Highway and Urban Environment Symposium (eds Morrison, G. M. & Rauch, S.) 235–247 (Springer, 2007).
Dechesne, M., Barraud, S. & Bardin, J. P. Spatial distribution of pollution in an urban stormwater infiltration basin. J. Contam. Hydrol. 72 , 189–205 (2004).
Dierkes, C. & Geiger, W. F. Pollution retention capabilities of roadside soils. Water Sci. Technol. 39 , 201–208 (1999).
Sauvé, S., Mcbride, M. B., Norvell, W. A. & Hendershot, W. H. Copper solubility and speciation of in situ contaminated soils: effects of copper level, pH and organic matter. Water Air Soil Pollut. 100 , 133–149 (1997).
Sauvé, S., Manna, S., Turmel, M. C., Roy, A. G. & Courchesne, F. Solid-solution partitioning of Cd, Cu, Ni, Pb, and Zn in the organic horizons of a forest soil. Environ. Sci. Technol. 37 , 5191–5196 (2003).
El-Mufleh, A. et al. Distribution of PAHs and trace metals in urban stormwater sediments: combination of density fractionation, mineralogy and microanalysis. Environ. Sci. Pollut. Res. 21 , 9764–9776 (2014).
Rostvall, A. et al. Removal of pharmaceuticals, perfluoroalkyl substances and other micropollutants from wastewater using lignite, Xylit, sand, granular activated carbon (GAC) and GAC+Polonite® in column tests – role of physicochemical properties. Water Res. 137 , 97–106 (2018).
Paredes, L., Fernandez-Fontaina, E., Lema, J. M., Omil, F. & Carballa, M. Understanding the fate of organic micropollutants in sand and granular activated carbon biofiltration systems. Sci. Total Environ. 551–552 , 640–648 (2016).
FAO, ITPS, GSBI, SCBD & EC. State of knowledge of soil biodiversity - status, challenges and potentialities. FAO https://doi.org/10.4060/cb1928en (2020).
Tietz, A., Langergraber, G., Watzinger, A., Haberl, R. & Kirschner, A. K. T. Bacterial carbon utilization in vertical subsurface flow constructed wetlands. Water Res. 42 , 1622–1634 (2008).
Weil, R. R. & Brady, N. C. The Nature and Properties of Soils 15th edn (Pearson Education, 2016).
Usman, K., Al-Ghouti, M. A. & Abu-Dieyeh, M. H. The assessment of cadmium, chromium, copper, and nickel tolerance and bioaccumulation by shrub plant Tetraena qataranse . Sci. Rep. 9 , 1–11 (2019).
Nuel, M., Laurent, J., Bois, P., Heintz, D. & Wanko, A. Seasonal and ageing effect on the behaviour of 86 drugs in a full-scale surface treatment wetland: removal efficiencies and distribution in plants and sediments. Sci. Total Environ. 615 , 1099–1109 (2018).
FAO. World Reference Base For Soil Resources 2014. International Soil Classification System For Naming Soils And Creating Legends For Soil Maps . World Soil Resources Report No. 106 (2014).
Villette, C. et al. In situ localization of micropollutants and associated stress response in Populus nigra leaves. Environ. Int. 126 , 523–532 (2019).
Schymanski, E. L. et al. Identifying small molecules via high resolution mass spectrometry: communicating confidence. Environ. Sci. Technol. 48 , 2097–2098 (2014).
Boleda, M. R., Galceran, M. T. & Ventura, F. Validation and uncertainty estimation of a multiresidue method for pharmaceuticals in surface and treated waters by liquid chromatography-tandem mass spectrometry. J. Chromatogr. A 1286 , 146–158 (2013).
Barupal, D. K. & Fiehn, O. Chemical similarity enrichment analysis (ChemRICH) as alternative to biochemical pathway mapping for metabolomic datasets. Sci. Rep. 7 , 1–11 (2017).
Download references
Acknowledgements
We acknowledge Martine Trautmann for the physico-chemical analysis of the soil samples. We also acknowledge all the collaborators who were involved in the project, from the Eurométropole de Strasbourg (Michaël Malfroy-camine, Yan Dabrowski, Maxime Pomies, Mikaël Magnin), Agence de l’Eau Rhin-Meuse (Roger Flutsch, Nicolas Venandet), ENGEES, APRONA and IBMP. We acknowledge Martin Fischer for his technical support on the project. This project was funded by a grant from the Agence de l’Eau Rhin-Meuse (AERM) and the Eurométropole de Strasbourg (grant number 165414).
Author information
Authors and affiliations.
Plant Imaging & Mass Spectrometry (PIMS), Institut de biologie moléculaire des plantes, CNRS, Université de Strasbourg, Strasbourg, France
Loïc Maurer, Julie Zumsteg, Dimitri Heintz & Claire Villette
CNRS, ENGEES, ICube UMR 7357, University of Strasbourg, Strasbourg, France
Loïc Maurer, Adrien Wanko & Claire Villette
Laboratoire d’étude des eaux, Ecole nationale du génie de l’eau et de l’environnement de Strasbourg, Strasbourg, France
Carole Lutz & Marie Pierre Ottermatte
You can also search for this author in PubMed Google Scholar
Contributions
L.M. sampled the soil, prepared the samples, analysed the data, prepared the figures and discussed the manuscript. C.V. designed the experiment, injected the samples for LC-HRMS, analysed the data, prepared the figures and wrote the manuscript. J.Z. injected the samples for GC-MS/MS and discussed the results. C.L. and M.P.O. performed the metals and chlorides analysis. A.W. discussed the results and manuscript. D.H designed the experiment, discussed the results and the manuscript.
Corresponding author
Correspondence to Claire Villette .
Ethics declarations
Competing interests.
The authors declare no competing interests.
Additional information
Publisher’s note Springer Nature remains neutral with regard to jurisdictional claims in published maps and institutional affiliations.
Supplementary information
Supplementary information, supplementary data 1, supplementary data 2, rights and permissions.
Open Access This article is licensed under a Creative Commons Attribution 4.0 International License, which permits use, sharing, adaptation, distribution and reproduction in any medium or format, as long as you give appropriate credit to the original author(s) and the source, provide a link to the Creative Commons license, and indicate if changes were made. The images or other third party material in this article are included in the article’s Creative Commons license, unless indicated otherwise in a credit line to the material. If material is not included in the article’s Creative Commons license and your intended use is not permitted by statutory regulation or exceeds the permitted use, you will need to obtain permission directly from the copyright holder. To view a copy of this license, visit http://creativecommons.org/licenses/by/4.0/ .
Reprints and permissions
About this article
Cite this article.
Maurer, L., Zumsteg, J., Lutz, C. et al. Towards a model for road runoff infiltration management. npj Clean Water 4 , 44 (2021). https://doi.org/10.1038/s41545-021-00136-z
Download citation
Received : 23 April 2021
Accepted : 20 August 2021
Published : 10 September 2021
DOI : https://doi.org/10.1038/s41545-021-00136-z
Share this article
Anyone you share the following link with will be able to read this content:
Sorry, a shareable link is not currently available for this article.
Provided by the Springer Nature SharedIt content-sharing initiative
This article is cited by
Mass spectrometry imaging for biosolids characterization to assess ecological or health risks before reuse.
- Claire Villette
- Loïc Maurer
- Dimitri Heintz
Nature Communications (2023)
Quick links
- Explore articles by subject
- Guide to authors
- Editorial policies
Sign up for the Nature Briefing: Anthropocene newsletter — what matters in anthropocene research, free to your inbox weekly.

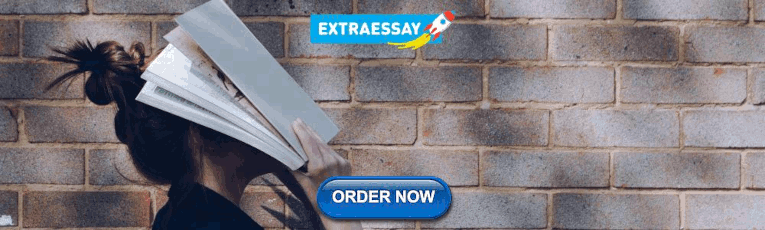
COMMENTS
PhD Thesis, Technical University . of Denmark, DTU Library. Citations (7) ... (SCMs), sustainable urban drainage systems, water sensitive urban design, green infrastructure (GI), and low impact ...
Best management practices (BMPs), which are also referred to as sustainable urban drainage systems (SUDS), are popular structural methods to help reduce urban floods and increase UDS sustainability. Scientists believe that storm-water management may be performed at three levels: site level, urban drainage basin level, and the whole river basin ...
Ph.D. Thesis. Department of Environmental Engineering, Tech nical University of De nmark, Lyngby, Denmark, ... Sustainable urban drainage systems (SUDS), e.g., permeable pavements, detention ponds ...
Climate change is arguably the greatest challenge facing cities today. Its severe consequences have created the need for sustainable urban planning. In this regard, Sustainable Drainage Systems (SuDS) have contributed in recent years to alleviating environmental problems caused by soil sealing and enhancing the resilience of cities to climate change. However, in most cases, the level of ...
Instead of focusing on 'end-of-pipe' or 'at the point of the problem' solutions as is the case with many purely 'grey' infrastructure solutions, sustainable urban drainage systems aim to slow down and reduce the quantity of surface water runoff in an area in order to minimize downstream flood risk and reduce the risk of resultant diffuse pollution to urban water bodies (Rose and ...
Climate change and urbanization are converging to challenge city drainage infrastructure due to their adverse impacts on precipitation extremes and the environment of urban areas. Sustainable drainage systems have gained growing public interest in recent years, as a result of its positive effects on water quality and quantity issues and additional recreational amenities perceived in the urban ...
Sustainable Urban Drainage Systems A thesis submitted to the University of Manchester for the degree of Doctor of Philosophy in the Faculty of Science and Engineering 2021 Omid Seyedashraf School of Mechanical, Aerospace and Civil Engineering . 2 Contents
Sustainable urban drainage systems (SUDS), or urban green infrastructure for stormwater control, emerged for more sustainable management of runoff in cities and provide other benefits such as urban mitigation and adaptation to climate change. Research in Spain began a little over twenty years ago, which was later than in other European countries, and it began in a heterogeneous way, both in ...
Student thesis: Phd. Abstract Sustainable urban drainage systems (SuDS) are multi-functional nature-based solutions to stormwater management problems. During the past years, there has been a growing interest in the application of multi-objective optimisation methods to facilitate design of SuDS. This has allowed decision-makers to select ...
Climate change and urbanization are converging to challenge city drainage infrastructure due to their adverse impacts on precipitation extremes and the environment of urban areas. Sustainable drainage systems have gained growing public interest in recent years, as a result of its positive effects on water quality and quantity issues and additional recreational amenities perceived in the urban ...
1. Storm-water management through sustainable urban drainage systems and the theory on planning systems (Urban) Pluvial flooding risk is an intrinsically anthropogenic concept as it is identified in impacts and damages caused on urban areas (communities, environment and economics) and because its understanding is strictly related to the individuals' perception (O'Neill, Brereton, Shahumyan ...
The research presented in this thesis addressed the performance of Sustainable Urban Drainage Systems (SUDS) at three sites in Scotland - a porous paved car park and two swales. It is the first research to provide results for such systems in the UK and also the first direct comparison between SUDS and traditional systems in situ.
Our research degrees. You have the option of studying an MSc by Research, an MPhil or a PhD. When deciding which degree is right for you, you should consider the level of commitment required, the duration, cost and your career aspirations. That being said, our research degrees are flexible. You can begin an MSc or an MPhil and then seek to ...
We focused on the barriers to the implementation of enabling environments for sustainable urban drainage systems (SUDS) in Belarus, Russia, and Ukraine. Based on interviews and desktop research, we analyzed overall framework conditions in these countries as well as implementation practices in three cities.
Consult the top 50 dissertations / theses for your research on the topic 'Sustainable urban drainage system.'. Next to every source in the list of references, there is an 'Add to bibliography' button. Press on it, and we will generate automatically the bibliographic reference to the chosen work in the citation style you need: APA, MLA, Harvard ...
Recently, urban areas have experienced frequent, large-scale flooding, a situation that has been aggravated by climate change. This study aims to improve the urban drainage system to facilitate climate change adaptation. A methodology and a series of mitigation strategies are presented to efficiently improve the urban drainage system in light of climate change. In addition, we assess the ...
The thesis points towards an integrated framework for urban drainage adaptation design considering climate change effects and adaptation benefits and costs. The case studies show how the proposed framework can be utilized to manage the anticipated climate change risks in a cost-effective way under different circumstances.
drainage system; flooding 1 Introduction An urban drainage system (UDS) is a key infrastruc-ture to control urban flooding. Since UDSs are exposed to uncertain future drivers such as climate change and urban development, adapting them to such changes to improve reliability is of great importance. During rainy seasons, some urban areas may be ...
In recent years, concepts of NBS for storm-water management have appeared under many terms worldwide, i.e., Sustainable Urban Drainage System, Low Impact Developments in US, Blue-Green Cities in ...
Abstract. In human society, there is a demand for sustainable solutions for water preservation and efficient treatment systems. An important water reservoir is road runoff defined as rainwater ...
Urban drainage systems face intrinsic constraints related to the deterioration of infrastructure, the interaction between systems, and increasing requirements and stresses that lower the quality of provided services. Furthermore, climate change and the need for the efficient use of resources are providing additional pressures that cannot be addressed solely with "Business-as-usual" solutions.
1. Trends in urban development. Urban areas are coming under ever increasing strain.The population of urban areas has been steadily increasing over the last decades. For instance, as is shown in. Figure 1. , the proportion of urban dwellers has increased from 30% in 1950 to 55% in 2018 and is projected to rise to 68% by 2050.
With the increasing intensity, frequency and duration of heat waves, adaptation measures are becoming increasingly relevant and are moving up the agenda of decision-makers. In particular, urban areas require effective solutions due to the urban heat island effect and the increasing number of urban dwellers, including highly vulnerable social groups, such as people with low income or who lack ...