- College of Arts & Sciences
- Graduate Division
- College of Liberal and Professional Studies

- Research - Plant Biology
Humankind depends on plant growth and productivity not only for human sustenance, but also for alternatives to fossil fuel or nuclear energy. In addition, plants are important for climate stability, and are a key resource for discovering new macromolecules that have applications in medicine and other important fields. Here at the Department of Biology, plant Researchers make use of reference species as well as ecologically important plant species employing both high throughput analyses as well as classical tools to address these questions and to generate basic knowledge about plant form and function.

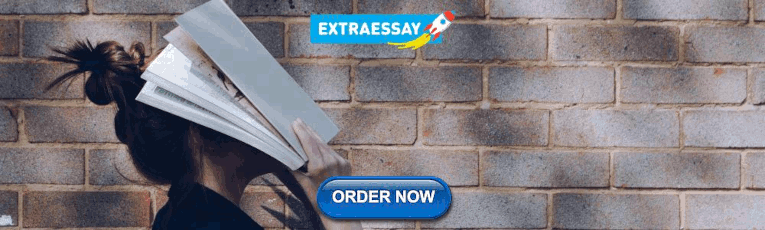
Kimberly Gallagher
Professor of Biology Chair of Biology
[email protected] 215-746-3605
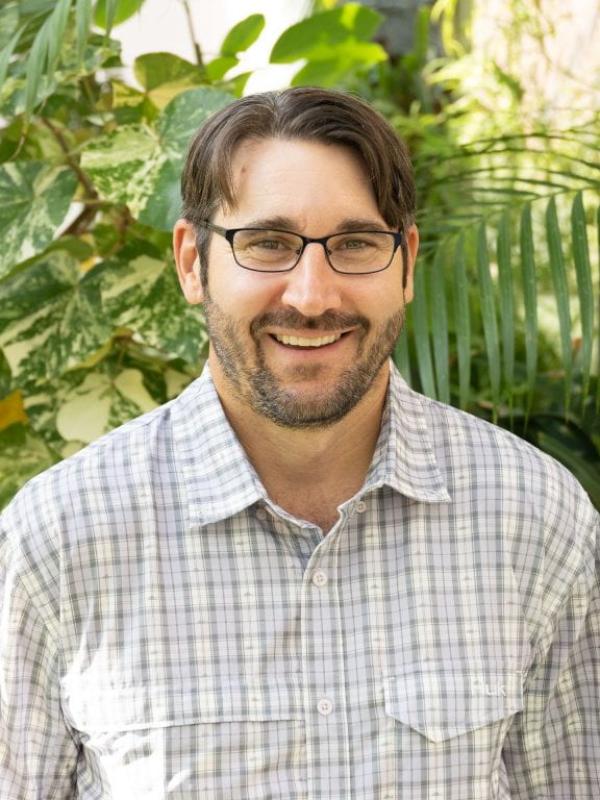
Brian D. Gregory
Professor of Biology
[email protected] 215-746-4398
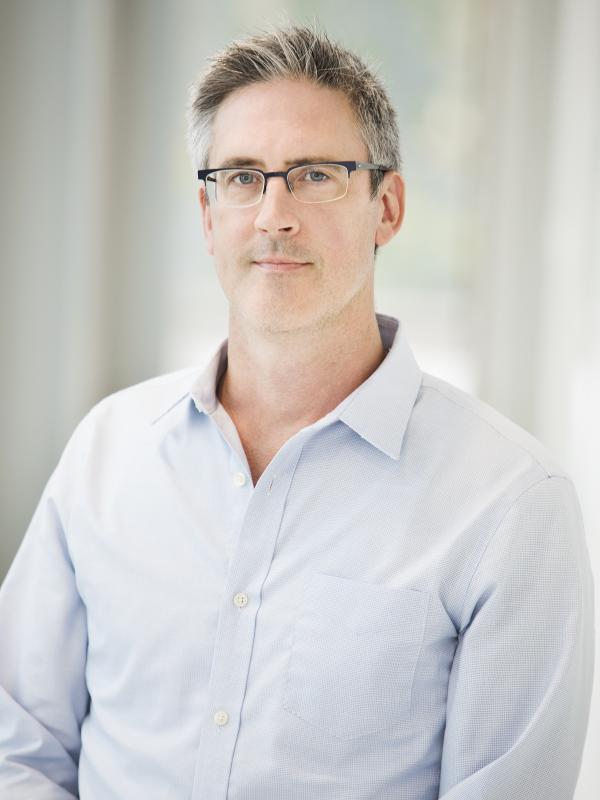
Brent Helliker
[email protected] 215-746-6217
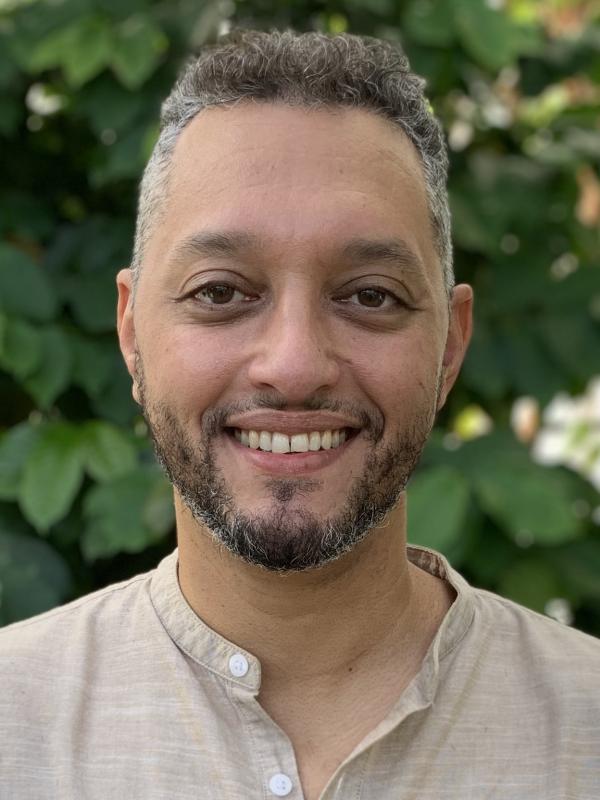
Aman Husbands
Mitchell J. Blutt and Margo Krody Blutt Presidential Assistant Professor of Biology
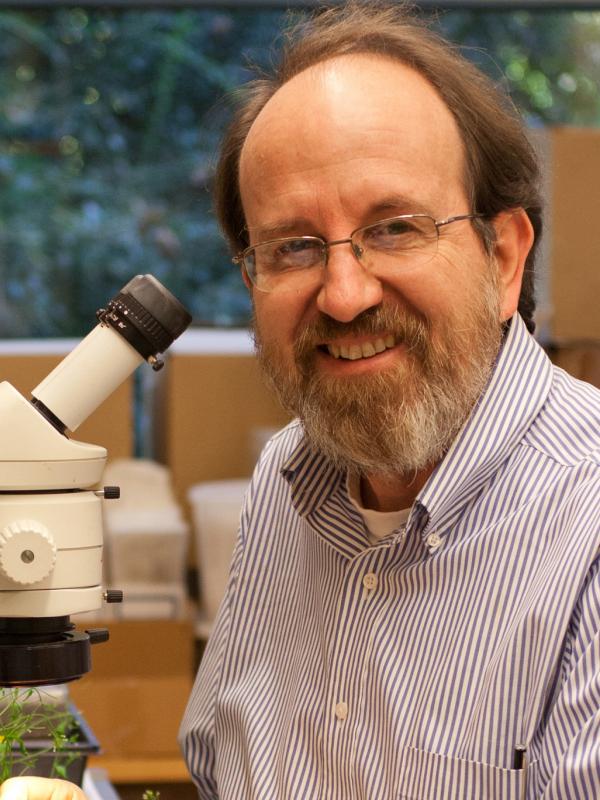
Scott Poethig
John H. and Margaret B. Fassitt Professor
[email protected] 215-898-8915

Doris Wagner
DiMaura Professor of Biology

Corlett Wood
Assistant Professor of Biology
Journal of Plant Research
- Encourages work based on unique approaches and unprecedented findings.
- Welcomes interdisciplinary studies and newly developing areas of basic plant biology.
- Papers should be hypothesis-driven, not purely descriptive.
- Manuscripts in applied plant sciences accepted only if they contribute to basic plant sciences.
- Covers a broad range of disciplines including taxonomy, phylogeny, evolutionary biology, and more.
- Maki Katsuhara
Societies and partnerships
Latest issue
Volume 137, Issue 3
Including the JPR Symposium on Update of Phosphate Transport Regulations
Latest articles
2024 awards in the journal of plant research, overexpression of sweetpotato glutamylcysteine synthetase ( ibgcs ) in arabidopsis confers tolerance to drought and salt stresses.
- Wenbin Wang
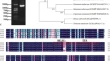
Plastome structure, phylogeny and evolution of plastid genes in Reevesia (Helicteroideae, Malvaceae)
- Li-Yang Geng
- Tian-Yi Jiang
- Yun-Fei Deng
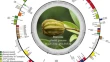
Update of phosphate transport regulations
- Satomi Kanno
- Laurent Nussaume
Modulation of plant immunity and biotic interactions under phosphate deficiency
- Kanako Inoue
- Natsuki Tsuchida
- Yusuke Saijo
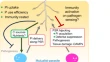
Journal updates
Journal information.
The Journal of Plant Research is the official journal of The Botanical Society of Japan. Society information, Journal of Plant Research Paper Award.
JPR SPECIAL COLLECTIONS & JPR SYMPOSIA
The JPR Special Collection (formerly Virtual Issue) puts together articles previously published in various issues, all centered around a specific topic. The JPR Symposium is a special feature published in a single issue.
COVER GALLERY
Each issue of the Journal of Plant Research has a different cover. In this cover gallery, you can browse the covers of past issues as well as their captions.
JOURNAL OF PLANT RESEARCH AWARDS
The Editorial Committee of the Botanical Society of Japan annually select papers for the Journal of Plant Research 'Best Paper Award' and 'Most-Cited Paper Award'.
Journal information
- Astrophysics Data System (ADS)
- Biological Abstracts
- CAB Abstracts
- Chemical Abstracts Service (CAS)
- Current Contents/Agriculture, Biology & Environmental Sciences
- Google Scholar
- Japanese Science and Technology Agency (JST)
- OCLC WorldCat Discovery Service
- Pathway Studio
- Science Citation Index Expanded (SCIE)
- TD Net Discovery Service
- UGC-CARE List (India)
- Zoological Record
Rights and permissions
Editorial policies
© The Botanical Society of Japan
- Find a journal
- Publish with us
- Track your research
- Utility Menu

Plant Biology Initiative at Harvard University
Pbi-logo.png.
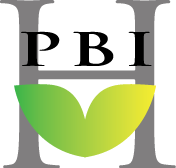
The Plant Biology Initiative at Harvard University
Harvard University is home to a wide range of plant biologists, primarily situated in the Department of Organismic and Evolutionary Biology and the research programs of the Arnold Arboretum and the Harvard Forest . These researchers apply a diverse array of tools and approaches to investigate questions in plant evolution, ecology, physiology, development and molecular biology. Our program takes full advantage of the exceptional resources of Harvard University, which include ~5,000,000 preserved specimens of the Harvard University Herbaria (HUH), the Botanical Libraries of the HUH, the living collections of the Arnold Arboretum , the 3500 acre Harvard Forest , and the resources and expertise of the FAS Center for Systems Biology .
PBI in the News
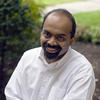
L. Mahadevan elected Fellow of American Academy of Arts and Sciences

Researchers discover root exudates have surprising and counterintuitive impact on soil carbon storage
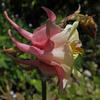
New study reveals novel interactions in the key processes that establish floral morphology
Save the date, the 17th annual plant biology initiative symposium " root-microbe interactions in a changing world " will take place may 6 & 7, 2024.
Register today!
For those unable to attend, you can livestream the talks on the OEB YouTube Channel
Plant Biology

Dominique Bergmann

Joseph A Berry

Adrien Burlacot
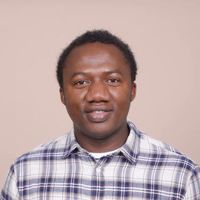
Barnabas Daru

José R. Dinneny
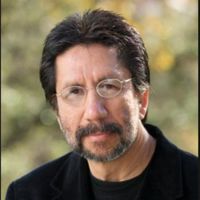
Rodolfo Dirzo

David Ehrhardt

Moises Exposito-Alonso

Chris Field
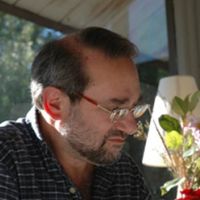
Arthur Grossman

Andrew Leslie
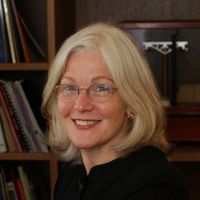
Sharon R. Long

Erin Mordecai

Mary Beth Mudgett

Virginia Walbot
Jump to navigation

- Alumni and Friends
- For Postdocs
- For the Department
- For the Faculty
- Parking Permits
- Room Reservations
- Equipment Check-Out
- Graduate Students
- Research Staff
- Department Staff
- Research Focus
- Research Services
- Graduate Group in Microbiology
- Cooperative Extension
- Undergraduate Majors
Graduate Programs
- Equity in Action
- Campus Resources
- CLEAR: Science Communications
- Resources for the Scientific Community
- Endowed Lectures
Search form
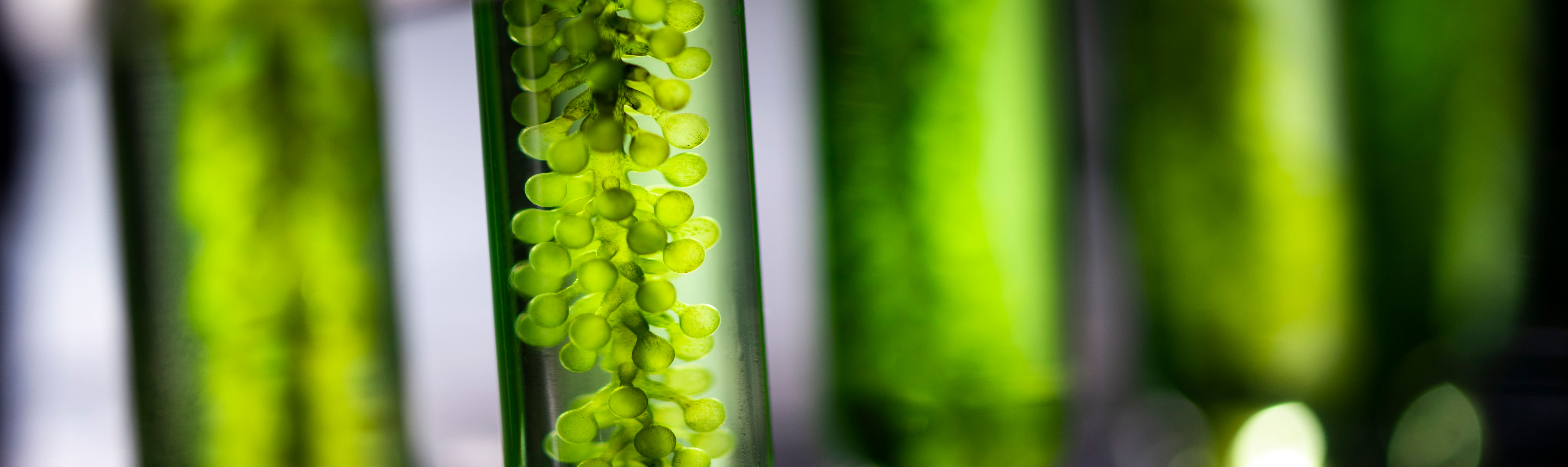
Upcoming Events
Our graduate programs are designed to enhance student preparation, engagement, and research training. Students experience the excitement of discovery in fundamental and applied research, and the satisfaction of teaching in the classroom.
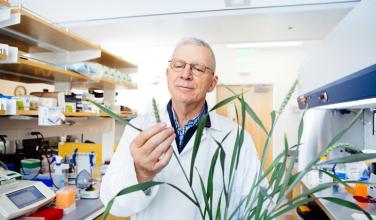
A half-century of plant immunity breakthroughs
Professor Emerit Brian Staskawicz and co-authors break down the last half-century of breakthroughs in the study of plant immunity in the 50th-anniversary issue of Cell .
More Headlines
Patrick shih named 2024 spark award recipient, advancing scientific understanding of calcium signaling, student spotlight: trinity reimer, karine gibbs on microbiology and mentorship.
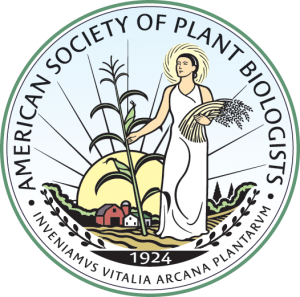
ASPB, Stronger Together.
ASPB is a professional society devoted to the advancement of the plant sciences.

ASPB membership dues provide value and help ASPB provide many resources to the global plant science community.
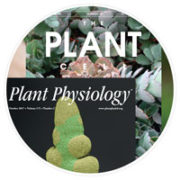
ASPB’s publishing program is dedicated to supporting, advancing, and sustaining international plant science research.
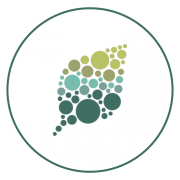
ASPB developed Plantae to address the need for a community and knowledge foundation for all plant scientists.
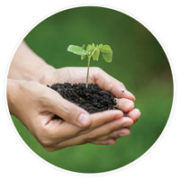
Help ASPB support students and the development of innovative programs and activities at ASPB.
2024 Election Slate
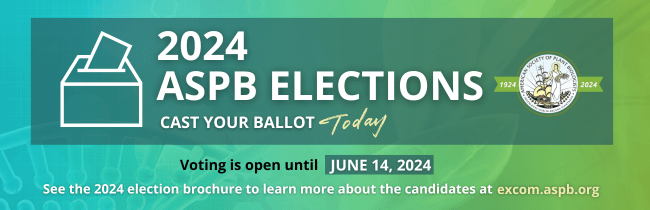
Plant Biology 2024
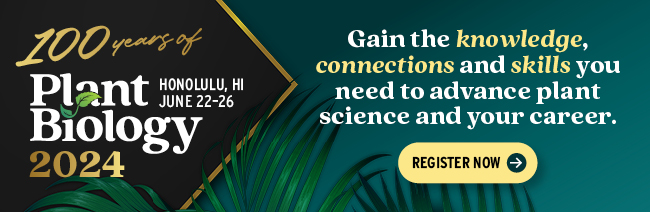
The Plant Cell Webinars
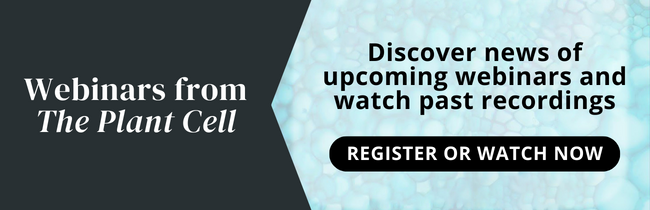
Plant Physiology Webinars
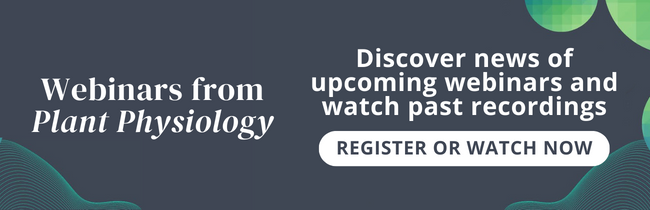
2024 Focus Issue on Translational Reseach
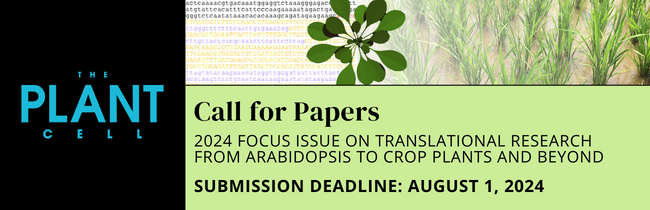
News & Updates
- Plant Physiology Focus Issue Webinar: Vegetable Crops, June 5, 2024 Plant Physiology Webinar: Vegetable Crops Celebrating the June 2024 Focus Issue on Vegetable Crops Wednesday June 5, 2025, 7AM GMT,... Read more Source: Blog Feed Published on: 2024-05-21
- Plant Physiology is recruiting Assistant Features Editors to start in 2025 and 2026 By Yunde Zhao (Plant Physiology EIC) and Mary Williams (Plant Physiology Features Editor) We started the Plant Physiology Assistant Features... Read more Source: Blog Feed Published on: 2024-05-16
- 25 Inspiring Women in Plant Biology In March, the ASPB Women in Plant Biology Committee asked you to nominate remarkable women who shape our field for... Read more Source: Blog Feed Published on: 2024-05-15
- ASPB Webinar April 24: What I Wish I Had Known When I Started Grad School (2024 Edition) A Panel Discussion by the ASPB Early Career Plant Scientists (ECPS) Section April 24, 2024 1 PM CST The ASPB... Read more Source: Blog Feed Published on: 2024-04-10
- Congratulations to ASPB’s 2024 Award Recipients The American Society of Plant Biologists (ASPB) is pleased to announce the recipients of its 2024 awards, which honor distinction... Read more Source: Blog Feed Published on: 2024-04-08
Upcoming Events
Visit the Global Plant Science Events Calendar on Plantae.org
Become and Advocate
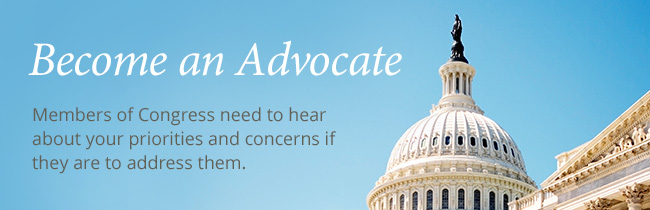
Early Career Professional Rep to Committees
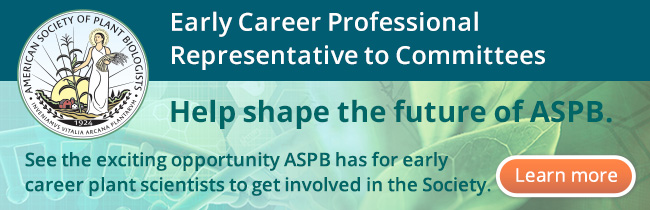
Give your students roots
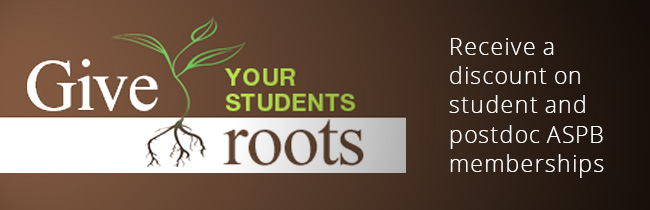
SSPA - Scientific Society Publisher Alliance
Plant cell translational research, american society of plant biologists is a 501(c)3 and donations are tax-deductible to the extent allowed by law. ein: 53-0258999.
- Manage your Membership
- Join an ASPB Section
- Visit our Store
- Sponsorship & Advertising
- Make a Donation
- Read the Plant Science Today Blog
Member Services
- (301) 251-0560
Awards & Funding
- Apply for Grants & Travel Awards
Meetings & Events
- Meeting Management Services
- Plant Synthetic Biology 2021
- Plant Biology Meeting
Publications & News
- ASPB Journals
- Read The Plant Cell Blog
- Read the Plant Physiology Blog
- Submit an Article
- Read the ASPB News
- Get News & Updates
- Check out The Signal
About Plantae
- Join Plantae
- Subscribe to the Plant Science Research Weekly
- Search for Careers & Internships
- Listen to Plantae Podcasts
- Submit your Science Event to our Calendar
- *All Plantae content is licensed under a Creative Commons A-NC 2.0 License

An official website of the United States government
The .gov means it's official. Federal government websites often end in .gov or .mil. Before sharing sensitive information, make sure you're on a federal government site.
The site is secure. The https:// ensures that you are connecting to the official website and that any information you provide is encrypted and transmitted securely.
- Publications
- Account settings
- Browse Titles
NCBI Bookshelf. A service of the National Library of Medicine, National Institutes of Health.
National Research Council (US) Committee on Examination of Plant Science Research Programs in the United States. Plant Biology Research and Training for the 21st Century. Washington (DC): National Academies Press (US); 1992.

Plant Biology Research and Training for the 21st Century.
- Hardcopy Version at National Academies Press
1 Why Plant-Biology Research Today?
Throughout human history, plants have been the object of pervasive and at times dominant artistic and intellectual interest. Plants were important subjects from the earliest study of life processes, and they were central to scientific study in the nineteenth and early twentieth centuries.
Good reasons remain to study the basic life processes of plants. Research on plants enriches our intellectual life and adds to our knowledge about other life processes. The results of research on plant systems also can teach us how to approach problems in agriculture, health, and the environment.
- Plants, Human Health, and Civilization
Our understanding of plant life underpins a vast range of activities and touches virtually every aspect of human life. From their origins, human civilizations have depended for their development and prosperity on their ability to manage plants and have sometimes fallen because of their failure to do so. Throughout history, plants have been collected, traded, selectively adapted for new environments, and bred for new combinations of traits. Plants have been manipulated for use as food and fiber, and for many other, particularly aesthetic, purposes.
Modern civilization rests on the successful and sustained cultivation of plants and on the wise use of the biologic and physical resource base on which their cultivation depends. Our knowledge about the world around us is incomplete if we do not include plants in our discoveries, and it is distorted if we do not place sufficient emphasis on plant life. There are many compelling practical reasons also for society to invest in research about plants and to educate its citizens for careers in which knowledge about plants is important. From fundamental discoveries about plant life arise technologies and capabilities in a wide range of practical applications ( Figure 2 ).
Potential applications of plan-biology research.
- Plants and the Environment
Only higher plants and a few microorganisms can convert light energy from the sun into chemical energy. Photosynthetic organisms are at the center of the earth's hospitality to other life. Plants and photosynthetic bacteria gave rise to the earth's atmosphere. They are important in regulating climate and the chemical and biologic conditions of the soil and water. Photosynthetic plants are the source of the fossil fuels we are depleting today, and they provide the most readily harvested source of renewable energy for tomorrow. The primary atmospheric gas incorporated by plants in photosynthesis, carbon dioxide, is one of the major ''greenhouse'' gases. Plants regulate the carbon cycle of the biosphere. Plants, in part through their unique symbiotic relationships with microorganisms, also play a major role in regulating the partitioning of nitrogen between atmospheric and life processes. We will never fully understand the global environment—or have a serious hope of successfully managing it in the face of explosive population growth—until we have a much more comprehensive understanding of plants, their cellular processes, and their ecology and population biology.
Plants are important in maintaining a healthy environment, for example, by controlling erosion and water pollution, and by helping to reduce air pollution. They improve the environment for human activities everywhere—from indoor spaces to vast wilderness areas.
The role of terrestrial plants and marine phytoplankton in maintaining an environment suitable for human habitation is inadequately appreciated, but there is a growing recognition of the urgent need to illuminate the role of plants. The accumulated effects of more than a century of industrial activity, explosive population growth, severe shifts in land use, and other effects of human use of the earth show that human activities can overpower the buffering effects of the natural processes that regulate global climate. The health and wellbeing of the human race could well rest on our achieving a better understanding on which to base a more reasoned exploitation of plant life.
Unique and Scientifically Interesting Properties of Plants
Plants differ from animals in several important ways.
Development . The growth of a plant from an undifferentiated cell into a complete and mature organism requires only a few hormones. Moreover, plant cells are totipotent: It is possible to regenerate a whole plant from a single leaf or root cell. In contrast, specific cells (the germ line) of an animal in early development form the germ cells. Plants have no germ line in this sense and produce sexual organs and gametes from somatic tissue late in their development.
Biochemistry . Plants are virtually the sole source of new oxygen and carbohydrates on the planet. Light is harvested by unique organelles, the chloroplasts. Plants synthesize the 20 amino acids required for proteins, including the 10 amino acids that humans are unable to produce. Moreover, in a unique symbiotic relationship with some plants, microorganisms can fix atmospheric nitrogen for plant use in the synthesis of amino acids, proteins, and other compounds.
Physiology . Plants lack the major organ systems present in animals. Yet, their physiology permits them to respond to their environment. Instead of an immune system, they have inducible disease resistance mechanisms that enable them to make natural toxins against fungal and bacterial pathogens. Instead of a nervous system, they have a repertoire of receptors and pigments that allow them to respond to their environment. Instead of a muscular and skeletal system, they have a novel set of fibers for support. They are attached to their substrates, and they can move only by growing or by gaining or losing water.
Plants and Global Warming
Atmospheric modelers are trying to evaluate the effects of changes in carbon dioxide concentration on global weather patterns and temperature. Models that predict carbon dioxide uptake and water loss by leaves grown under different environmental conditions can make an important contribution to elucidating global climate change. Other plant research is needed to develop sensitive ways to determine how much of the light energy absorbed by a leaf is used for photosynthesis (for metabolism and growth) and how much is simply reradiated as heat. The efficiency with which plants use light can vary enormously in response to environmental variables, such as water stress, temperature, disease or insect damage, or fluctuations in the supply of nitrogen or phosphorus. Theoretical models are being rigorously tested, with a fair degree of success. In addition, remote-sensing techniques are being developed to evaluate the photosynthetic performance of whole plant communities in response to stress. Modeling and experimental studies promise the quantitative information required to put predictions of atmospheric change (or lack of it) on a sound basis.
- Plants in Agriculture, Medicine, and Industry
Macroscopic and microscopic plants form the first link in the terrestrial and aquatic food chains. Plants are thus at the heart of agriculture. Together with microorganisms and domesticated animals, plants provide the raw materials for our food and drink. Plants also provide many of the materials used in clothing and buildings. The application of basic knowledge about plants has made modern agriculture possible. For example, studies of the nutrient requirements of plants led to soil fertility management.
The Green Revolution was founded on fundamental knowledge gleaned from research in genetics and plant nutrition. Genetic manipulation is a powerful, proven method for improving the productivity, quality, and disease resistance of plants. Basic knowledge of genetic inheritance, defense responses, pathogen genetics, and population genetics will continue to yield improvements in the technology needed to secure a stable food supply.
Plants are critical to human health. They are the sole source of some of the essential amino acids, vitamins, and other nutrients in our diet. Research with plants was central to elucidating the role of vitamins in human health and disease: Plants high in ascorbic acid, such as peppers and citrus, prevent scurvy. Grains in the diet provide B vitamins. Many drugs were first discovered as plant products before methods for their synthesis were developed. Research on plants yielded cardiac glycosides (such as digitalis), a wide range of useful alkaloids (such as scopolamine, atropine, quinine, and ephedrine), dicoumarol, and many other drugs. Research on lower plants and agricultural soils yielded many antibiotics. Even today, more than 20 percent of all prescription drugs are derived from plants.
The chemical industry developed from the work of German scientists who learned to synthesize dyes from coal tar, a derivative of fossil plants, to replace the commonly used dyes derived from wild and cultivated plants. Now, the search has been reversed and plant-derived products are sought to replace harmful coal tar dyes. Modern industry and society continue to depend in many ways on chemical products derived from plants, such as soaps, detergents, rubber, paints, resins, plastics, adsorbents, and adhesives.
- Plants and the Origins of Modern Biology
Research with plants has strongly influenced the development of biology and has contributed to many important scientific advances. It was research with plants that led to the discovery of the rules of genetic inheritance (Gregor Mendel's peas), of the role of light in regulating the physiologic responses of higher organisms (phytochromes), of transposition of genetic elements (controlling elements in maize), and of the protein nature of enzymes (urease). Research with a plant virus contributed to the elucidation of the structure of DNA itself (X-ray diffraction with tobacco mosaic virus) and of the role of nucleic acids in the genetic material of all life forms.
These examples illustrate how the study of plants has affected biologic research for several generations. But how well equipped are we to deal with the opportunities and challenges that lie ahead? The techniques of modern biology, and in particular modern genetics, make many difficult problems in plant biology approachable. Before the era of recombinant DNA, the tools available for genetic studies of plants' development, metabolism, and environmental responsiveness were relatively crude. Now modern genetics offers new promise to the plant sciences. In some fields of modern biology, plants offer the preferred model system for fundamental and exploratory science through application of molecular genetic techniques. Scientists now can transfer genes easily among plant species, and because the genomes of some plant species are quite small they can be studied readily. Plants can be used to answer many general questions in biology in such diverse subdisciplines as development, metabolism, gene regulation, symbiosis, and chromosome structure.
It is not within the scope of this report to describe a research agenda for plant sciences. Other National Research Council reports have contained pertinent research agendas, for example, Investing in Research (NRC, 1989a), Opportunities in Biology (NRC, 1989b), and Forestry Research: A Mandate for Change (NRC, 1990).
In recent years, the scientific community has shown significantly increased interest in research with plants. The power of modern methods to answer important questions in plant biology has stimulated the interest of scientists in leading universities and other research institutions in the United States and abroad. Well-funded plant-biology laboratories here and elsewhere are making research contributions at the cutting edge of biology. This heightened interest has generated more worthy research proposals than public agencies can fund. An informal survey of the private sector in agricultural biotechnology indicates that in the late 1980s about $250 million (exclusive of development costs) each year was being spent on basic plant-biology research by companies whose work was primarily or exclusively with plants.
The fertility of modern plant-biology research is demonstrated in special issues of Science (November 16, 1990) and Cell (January 27, 1989). Developmental biology, cell-to-cell recognition, signal transduction, the molecular basis of disease, plant-microbe interactions, gene regulation, transposition, and photosynthesis are some of the areas covered in these issues. Several new plant journals have been launched recently; three leading examples are: The Plant Cell, The Plant Journal, and Plant Molecular Biology .
- Cite this Page National Research Council (US) Committee on Examination of Plant Science Research Programs in the United States. Plant Biology Research and Training for the 21st Century. Washington (DC): National Academies Press (US); 1992. 1, Why Plant-Biology Research Today?
- PDF version of this title (1.1M)
In this Page
Recent activity.
- Why Plant-Biology Research Today? - Plant Biology Research and Training for the ... Why Plant-Biology Research Today? - Plant Biology Research and Training for the 21st Century
Your browsing activity is empty.
Activity recording is turned off.
Turn recording back on
Connect with NLM
National Library of Medicine 8600 Rockville Pike Bethesda, MD 20894
Web Policies FOIA HHS Vulnerability Disclosure
Help Accessibility Careers

- Cornell University Home
- College of Agriculture & Life Sciences Home
School of Integrative Plant Science

Research: Plant Biology
The Plant Biology Section is home to some of the most influential plant science research in the world. The Section represents diverse intellectual interests and boasts a collaborative community culture. Including emeritus professors, there are three members of the National Academy of Sciences. Undergraduates, graduate students, and post-doctoral researchers can join field-leading faculty as they study a wide range of research areas and concentrations in plant sciences.
Research Areas in Plant Biology
Maureen Hanson 323 Biotechnology Building 607-254-4833
Gene expression in plant organelles; chloroplast movement and cell biology
Maria Harrison 405 Boyce Thompson Institute 607-254-6472
Arbuscular mycorrhizal symbiosis; plant phosphate transport and nutrition; root biology
Li Li 140 Robert Holley Center 607-255-5708
Regulation of plant secondary and micronutrient metabolism
Wojciech Pawlowski 401 Bradfield Hall 607-254-8745
Plant molecular biology; meiosis mechanisms of chromosome pairing and recombination
Eric Richards 125 Boyce Thompson Institute 607-254-4676
Epigenetics of Arabidopsis
Adrienne Roeder 239 Weill Hall 607-255-4467
Role of cell division and growth in plant development and patterning
Jocelyn Rose 331 Emerson Hall 607-255-4781
Structure, function and evolution of plant cell polymer networks; polysaccharide and cuticle matrices; cell wall biotechnology
Klaas van Wijk 332 Emerson Hall 607-255-3664
Chloroplast biogenesis; proteomics; mass spectrometry; Arabidopsis; biochemistry
Thomas Björkman Horticulture Science Cornell AgriTech
Developmental physiology; environmental regulation and root development
Susheng Gan 119 Plant Science 607-255-6088
Genomics, regulation and biotechnology of senescence; post-harvest biology
James Giovannoni 429 Boyce Thompson Institute 607-254-1259
Molecular and genetic analysis of tomato fruit ripening
Jian Hua 158 Emerson Hall 607-255-5554
Genetics of temperature responses in plants; regulation of plant defense responses
Gregory Martin 327 Boyce Thompson Institute 607-254-1208
Disease resistance; signal transduction; genomics; applications of genetic engineering
Michael Scanlon Plant Science 607-254-1156
Plant development; meristem function; evolution of leaf morphology
Chelsea Specht 502 Mann Library
Systematics and evolution of plants especially tropical monocots. Developmental evolution of plant traits associated with speciation and diversification.
Robert Turgeon 256 Plant Science 607-255-8395
Phloem transport; carbohydrate synthesis; phloem loading; plasmodesmata
Jeff J. Doyle 404 Mann Library 607-255-7972
Molecular systematics and evolution
Georg Jander 407 Boyce Thompson Institute 607-254-1365
Plant-insect interactions; plant amino acid biosynthesis; plant biochemistry
Susan McCouch 162 Emerson Hall 607-255-0420
Development and application of molecular tools for rice improvement
Martha Mutschler-Chu 303 Bradfield Hall 607-255-1660
Pest resistance and use of wild species in tomato and onion
Andre Kessler E445 Corson Hall 607-254-4219
Molecular, chemical ecology; plant-insect and multitrophic interactions; responses to herbivory
Gaurav Moghe 260 Emerson Hall Evolution of plant specialized metabolism, evolutionary genomics, computational biology, metabolic engineering for agriculture and medicine
Robert A. Raguso W355 Seeley G. Mudd Hall 607-254-4353
Reproductive ecology, plant volatile biosynthesis, floral evolution, plant signaling
Timothy Setter 235 Emerson 607-255-1319
Stress response, hormonal and sugar signaling; cell division; reproductive abortion
David Stern 301 Boyce Thompson Institute 607-254-1306
Regulation of plant organelle gene expression by nuclear factors
Olena Vatamaniuk 608 Bradfield Hall 607-255-8049
Heavy metal detoxification; proteome and metabolome responses to heavy metal toxicity
Adam Bogdanove 360 Plant Science (607) 255-7831
Bacterial blight and bacterial leaf streak of rice; transcription activator-like (TAL) effector proteins; genome editing and custom gene regulation
Gaurav Moghe 260 Emerson Hall
Evolution of plant specialized metabolism, evolutionary genomics, computational biology, metabolic engineering for agriculture and medicine
William Crepet 412B Mann Library 607-255-4075
Diversity, relationship and evolution of seed and flowering plants
Fay-Wei Li 607-254-1244 121 Boyce Thompson Institute
'Weird' biology -- evolutionary processes at the gene, genome, and microbiome levels that shape plant diversity.
Kevin Nixon 406B Mann Library 607-255-7975
Oak taxonomy; cladistics; computer programs; paleobotany, phylogeny of angiosperms
Thank you for visiting nature.com. You are using a browser version with limited support for CSS. To obtain the best experience, we recommend you use a more up to date browser (or turn off compatibility mode in Internet Explorer). In the meantime, to ensure continued support, we are displaying the site without styles and JavaScript.
- View all journals
Plant biotechnology articles from across Nature Portfolio
Plant biotechnology can be defined as the introduction of desirable traits into plants through genetic modification.
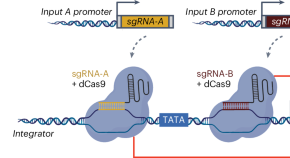
Advancing programmable gene expression in plants using CRISPRi-based Boolean gates
To advance the toolset for controlling plant gene expression, we developed a CRISPR interference-based platform for the construction of synthetic Boolean logic gates that is functional in multiple plant species. These genetic circuits are programmable and reversible in nature, which will enable spatiotemporal control of plant responses to dynamic cues.
Related Subjects
- Agricultural genetics
- Field trials
- Molecular engineering in plants
Latest Research and Reviews
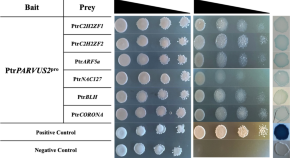
The transcriptional regulation of a putative hemicellulose gene, PtrPARVUS2 in poplar
- Heather D. Coleman
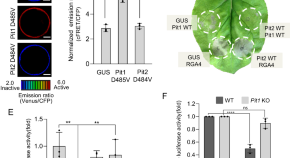
An NLR paralog Pit2 generated from tandem duplication of Pit1 fine-tunes Pit1 localization and function
The paralogous NLR proteins, Pit1 and Pit2, exhibit distinct functions in rice immunity, where Pit1 induces cell death on the plasma membrane and Pit2 inhibits this function by sequestering Pit1 to the cytosol.
- Yoji Kawano
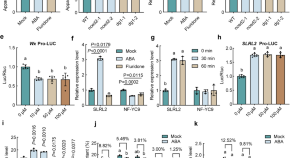
ABA-mediated regulation of rice grain quality and seed dormancy via the NF-YB1-SLRL2-bHLH144 Module
This study revealed an NF-YB1-SLRL2-bHLH144 regulatory module, centered on a key transcription factor SLRL2, that mediates the ABA-regulated amylose content in rice. Furthermore, SLRL2 is also involved in the regulation of rice dormancy
- Jin-Dong Wang
- Qian-Feng Li
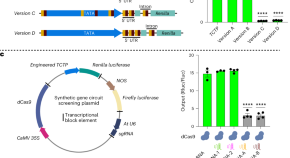
CRISPRi-based circuits to control gene expression in plants
Programmable and reversible CRISPRi-based genetic circuits function in a variety of plants.
- Muhammad Adil Khan
- Gabrielle Herring
- Ryan Lister
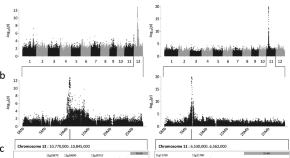
Genome-wide association analysis uncovers rice blast resistance alleles of Ptr and Pia
A GWAS on 500 genetically diverse rice accessions enables identification of an allelic series for the unusual Ptr rice blast resistance gene, and the Pia resistance locus.
- Julian R. Greenwood
- Vanica Lacorte-Apostol
- Simon G. Krattinger
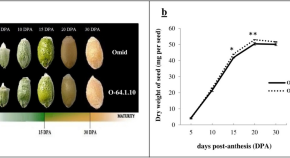
RNA-Seq transcriptome profiling of immature grain wheat is a technique for understanding comparative modeling of baking quality
- Hossein Ahmadi-Ochtapeh
- Hassan Soltanloo
- Vahid Shariati
News and Comment
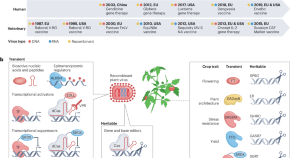
Engineering good viruses to improve crop performance
Viruses can be engineered to deliver nucleic acids, peptides and proteins for plant trait reprogramming. Building on market approvals and sales of recombinant virus-based biopharmaceuticals for veterinary and human medicine, similar innovations may be applied to agriculture for transient or heritable biodesign of crops with improved performance and sustainable production.
- Fabio Pasin
- Mireia Uranga
- Choon-Tak Kwon
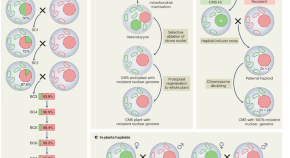
Haploids fast-track hybrid plant breeding
Two studies report the use of paternal haploids to enable one-step transfer of cytoplasmic male sterility in maize and broccoli, which resolves a key technical bottleneck in hybrid crop breeding.
- Ravi Maruthachalam
Feeding the future global population
Climate change is exacerbating challenges both for global food production and from its environmental impacts. Sustainable and socially responsible solutions for future world-wide food security are urgently needed.
Novel gene for herbicide resistance
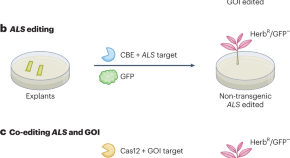
Blueprint for non-transgenic edited plants
A robust strategy to obtain edited crops without integration of a transgene is developed based on co-editing the ALS gene and a gene of interest.
- Jean-Luc Gallois
- Fabien Nogué
Quick links
- Explore articles by subject
- Guide to authors
- Editorial policies

- Search Menu
- Sign in through your institution
- Advance Articles
- Collections
- Focus Collections
- Teaching Tools in Plant Biology
- Browse by cover
- High-Impact Research
- Author Guidelines
- Quick and Simple Author Support
- Focus Issues Call for Papers
- Submission Site
- Open Access Options
- Self-Archiving Policy
- Why Publish with Us?
- About The Plant Cell
- About The American Society of Plant Biologists
- Editorial Board
- Advertising & Corporate Services
- Journals on Oxford Academic
- Books on Oxford Academic
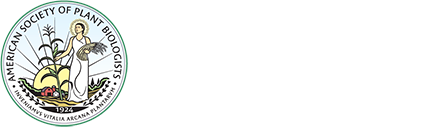
Browse issues

Cover image

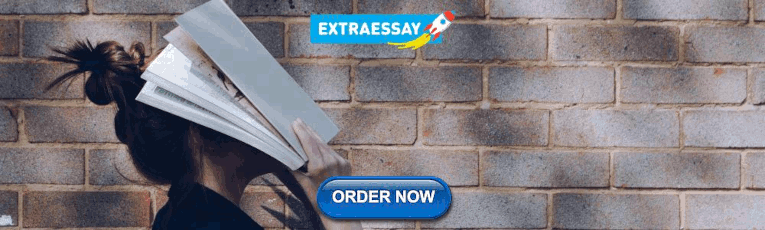
Volume 36, Issue 6, June 2024
Thank you to reviewers and editors for 2023.
- View article
From the archives: Tales from evolution—inflorescence diversity, gene duplication, and chromatin-mediated gene regulation
It takes two to tango: plant hosts influence bacterial effector function through post-translational modifications, find your way: building an atlas from transcription factor activities in the marchantia gemma, mining for phosphates: the myb73-1 - gdpd2 - ga2ox1 module as a hub to improve phosphate deficiency tolerance in soybean, the mrna puzzle: intron retention under stress, more pi, anyone interplay between brassinosteroid signaling and the phosphate starvation response, dicing dicer-like2 roles: identification of sirna-independent dicer-like2 functions, a compact topic: how ethylene controls crown root development in compacted soil, what is going on inside of phytochrome b photobodies.

CONSTANS, a HUB for all seasons: How photoperiod pervades plant physiology regulatory circuits

Breakthrough Report
Heat-induced sumoylation differentially affects bacterial effectors in plant cells.

Multiple effectors from the pathogen P. syringae are substrates of SUMOylation, which regulates distinct effectors differently in plant cells during bacterial infection under high temperatures.
- Supplementary data
Large-scale Biology
Pan-transcriptomic analysis reveals alternative splicing control of cold tolerance in rice.

Pan-transcriptomic analysis of rice under cold stress reveals alternative splicing control of cold tolerance.
The landscape of transcription factor promoter activity during vegetative development in Marchantia

A collection of promoters was systematically derived from transcription factor genes in Marchantia polymorpha and used to build a precise map of cell fates in vegetative propagules of the plant.
Integrative omics analysis elucidates the genetic basis underlying seed weight and oil content in soybean

Integration of multiple association studies reveals genetic loci, coexpression modules, and candidate genes that are significantly associated with simultaneous regulation of seed weight and oil content.
Research Articles
The myb73–gdpd2–ga2ox1 transcriptional regulatory module confers phosphate deficiency tolerance in soybean.

The major quantitative trait locus gene GmGDPD2 controls root and phosphorus efficiency traits and interacts with Myb73 and GA2ox1 to enhance yield traits.
Maternal nitric oxide homeostasis impacts female gametophyte development under optimal and stress conditions

Maternal nitric oxide homeostasis restricts auxin supply to the female gametophyte, disrupting its development under both optimal and stress conditions.
Arginine methylation of SM-LIKE PROTEIN 4 antagonistically affects alternative splicing during Arabidopsis stress responses

Arginine methylation of the spliceosome component SM-LIKE PROTEIN 4 by PROTEIN ARGININE METHYLTRANSFERASE5 fine-tunes alternative splicing of genes to control stress responses in Arabidopsis.
The chromatin remodeler ERCC6 and the histone chaperone NAP1 are involved in apurinic/apyrimidinic endonuclease-mediated DNA repair

APURINIC/APYRIMIDINIC ENDONUCLEASE REDOX PROTEIN recruits a chromatin remodeler and a histone chaperone to facilitate uracil N-glycosylase-triggered base excision repair.
Brassinosteroid-dependent phosphorylation of PHOSPHATE STARVATION RESPONSE2 reduces its DNA-binding ability in rice

Brassinosteroids modulate phosphate homeostasis by phosphorylating a serine residue of OsPHR2 through GSK2, thereby attenuating its DNA-binding activity.
Identification and functional characterization of conserved cis -regulatory elements responsible for early fruit development in cucurbit crops

Integrative analyses of conserved noncoding sequences and accessible chromatin regions provide insights into cis -regulatory elements and target sequences for fruit development in cucurbits.
Evidence for an RNAi-independent role of Arabidopsis DICER-LIKE2 in growth inhibition and basal antiviral resistance

Arabidopsis DICER-LIKE2 mediates growth repression and basal antiviral resistance in local infection contexts independently of the silencing activity of the small interfering RNAs it produces.
Nonspecific phospholipases C3 and C4 interact with PIN-FORMED2 to regulate growth and tropic responses in Arabidopsis

Nonspecific phospholipases NPC3 and NPC4 interact with the transporter PIN-FORMED2 and regulate auxin transport and signaling.
OPEN STOMATA 1 phosphorylates CYCLIC NUCLEOTIDE-GATED CHANNELs to trigger Ca 2+ signaling for abscisic acid-induced stomatal closure in Arabidopsis

Abscisic acid activates CYCLIC NUCLEOTIDE-GATED CHANNELs via OST1 kinase-mediated phosphorylation to elicit and regulate cytosolic Ca 2+ signaling for stomatal closure in Arabidopsis.
ENHANCER OF SHOOT REGENERATION1 promotes de novo root organogenesis after wounding in Arabidopsis leaf explants

The dual mode of action of ENHANCER OF SHOOT REGENERATION1, which occurs near wound sites of leaf explants, maximizes local auxin biosynthesis and de novo root organogenesis in Arabidopsis.
A gradient of the HD-Zip regulator Woolly regulates multicellular trichome morphogenesis in tomato

A gradient-dependent mechanism mediated by the HD-Zip IV regulator Woolly controls cell division and endoreduplication during tomato ( Solanum lycopersicum ) multicellular trichome morphogenesis.
The OsEIL1–OsWOX11 transcription factor module controls rice crown root development in response to soil compaction

The phytohormone ethylene fine-tunes rice crown root development by activating WUSCHEL-RELATED HOMEOBOX 11 expression in response to soil compaction.
H3K4me1 recruits DNA repair proteins in plants

DNA repair proteins preferentially repair regions with the epigenomic feature H3K4me1, resulting in mutation rate heterogeneity in plants.
Transcription factor FveMYB117a inhibits axillary bud outgrowth by regulating cytokinin homeostasis in woodland strawberry

An MYB transcription factor directly regulates cytokinin homeostasis to repress axillary bud growth and consequently affect crown formation in woodland strawberry.
Corrections
Correction to: h 2 o 2 -dependent oxidation of the transcription factor gmntl1 promotes salt tolerance in soybean, correction to: polyploid plants take cytonuclear perturbations in stride, email alerts.
- Recommend to Your Librarian
- Advertising & Corporate Services
- Awards & Funding
- Plant Science Today
- Plant Biology Meeting
- Meeting Management Services
- Plant Science Research Weekly
- Taproot: A Plantae Podcast
Affiliations
- Online ISSN 1532-298X
- Print ISSN 1040-4651
- Copyright © 2024 American Society of Plant Biologists
- About Oxford Academic
- Publish journals with us
- University press partners
- What we publish
- New features
- Open access
- Institutional account management
- Rights and permissions
- Get help with access
- Accessibility
- Advertising
- Media enquiries
- Oxford University Press
- Oxford Languages
- University of Oxford
Oxford University Press is a department of the University of Oxford. It furthers the University's objective of excellence in research, scholarship, and education by publishing worldwide
- Copyright © 2024 Oxford University Press
- Cookie settings
- Cookie policy
- Privacy policy
- Legal notice
This Feature Is Available To Subscribers Only
Sign In or Create an Account
This PDF is available to Subscribers Only
For full access to this pdf, sign in to an existing account, or purchase an annual subscription.
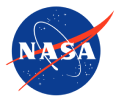
Plant Biology Program
Space Biology research helps us understand the fundamentals of plant growth by examining the very building blocks of plant life down to the molecular level: transcriptomics, genomics, proteomics, and metabolomics. To compare the effects of microgravity conditions on plants, we also conduct experiments on Earth using gravity or simulated microgravity ground controls at the Kennedy Space Center. We conduct our research on the ISS in conditions of microgravity to help us understand how to support astronauts aboard the ISS and on their long journey to Mars.
Crop Rotation: Food Production in Space
One of Space Biology’s major objectives is to understand how the spaceflight environment affects how plants grow and thrive. The basic research has allowed NASA scientists to grow edible plants in space that could be used as a source of fresh food by the crew on the ISS. Considering that every single thing that astronauts eat is freeze-dried and comes out of a shrink-wrapped package, being able to enjoy a fresh vegetable provides a healthy and much welcomed break from this routine. Already, edible romaine lettuce and cabbage has successfully been grown on the ISS. Soon, Mizuna and tomatoes will join the list of edible plants gown in pace.
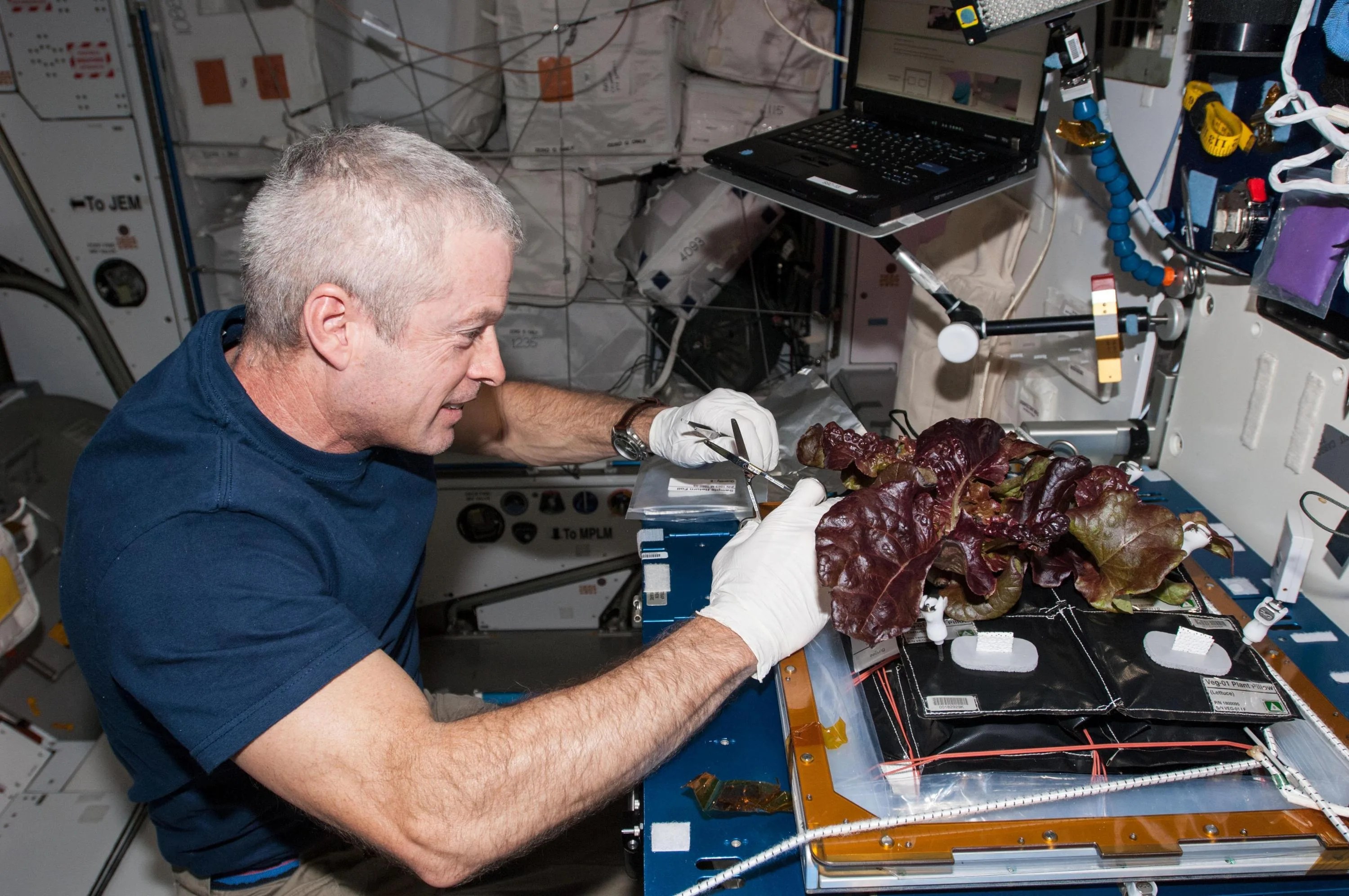
In the next year Space Biology will fly experiments to the ISS designed to test the growth of a variety of new plants its crew can eventually eat as they fly to the moon and Mars. To ensure the health of our astronauts, we’ll be examining the nutritional composition of plants grown in space, and looking at the microbiome of plants in orbit. This work may eventually lead to the production of a sustainable source of healthy food on long-duration space flights, which will help astronauts get the nutrition they need.
As we examine the impacts of spaceflight on plant biology, we ask the following:
- How does gravity affect plant growth, development & metabolism (e.g. photosynthesis, re- production, lignin formation, plant defense mechanisms)?
- Does the spaceflight environment cause alterations in plant/microbe interactions?
- How can horticultural approaches for sustained production of edible crops in space be both improved and implemented (especially as related to water and nutrient provision in the root zone)?
- What are the effects of chronic exposure to cosmic radiation on plants?
- How do plants sense and react to gravity and what are the molecular mechanisms involved?
More about our Plant Biology program:
Overview What We Study Experiments Hardware Publications
Department of Biological Sciences
- TTU Biology
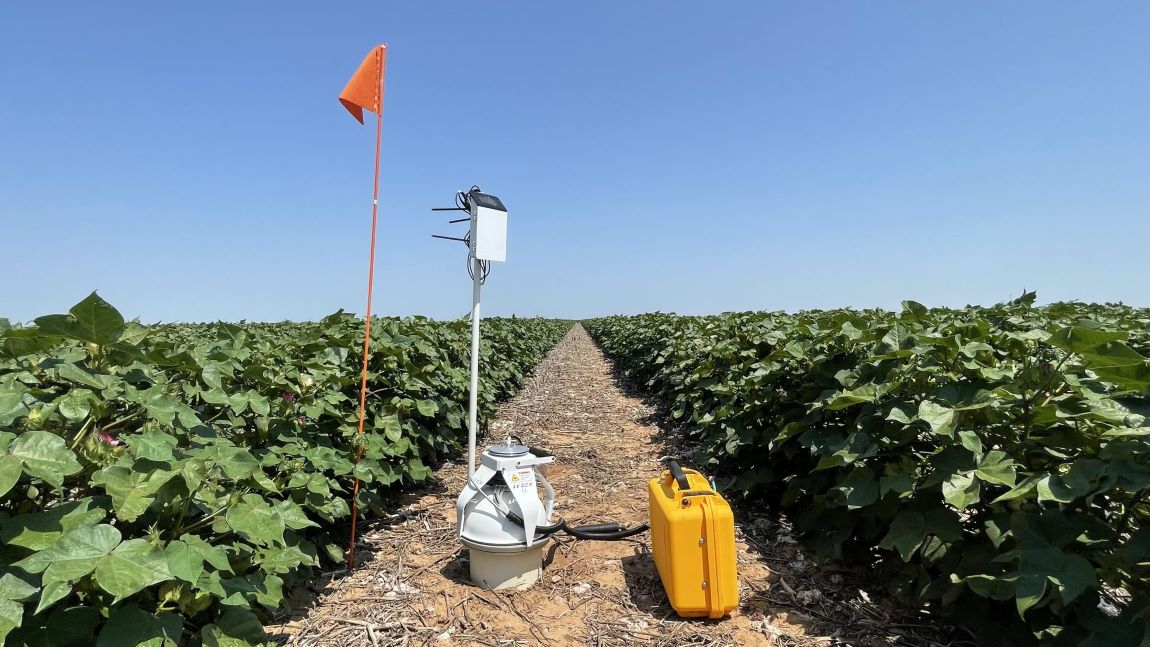
PLANT BIOLOGY
•Plant Molecular Biology •Small RNA-Mediated Processes in Plants •Plant Ecology and Evolution • Fire Ecology •Plant Molecular Biology •Plant Molecular Genetics •Plant Biotechnology •Soil Microbial Processes •Plant Physiology •Chihuahuan Desert •Phylogenomics •Plant Systematics •Bryophyte Evolution •Bioinformatics •Plant Population Genomics & Bioinformatics •Plant mating System Evolution •Soil/Sediment Microbial Ecology •Bioremediation •Restoration Ecology •Sustainability Sci ence
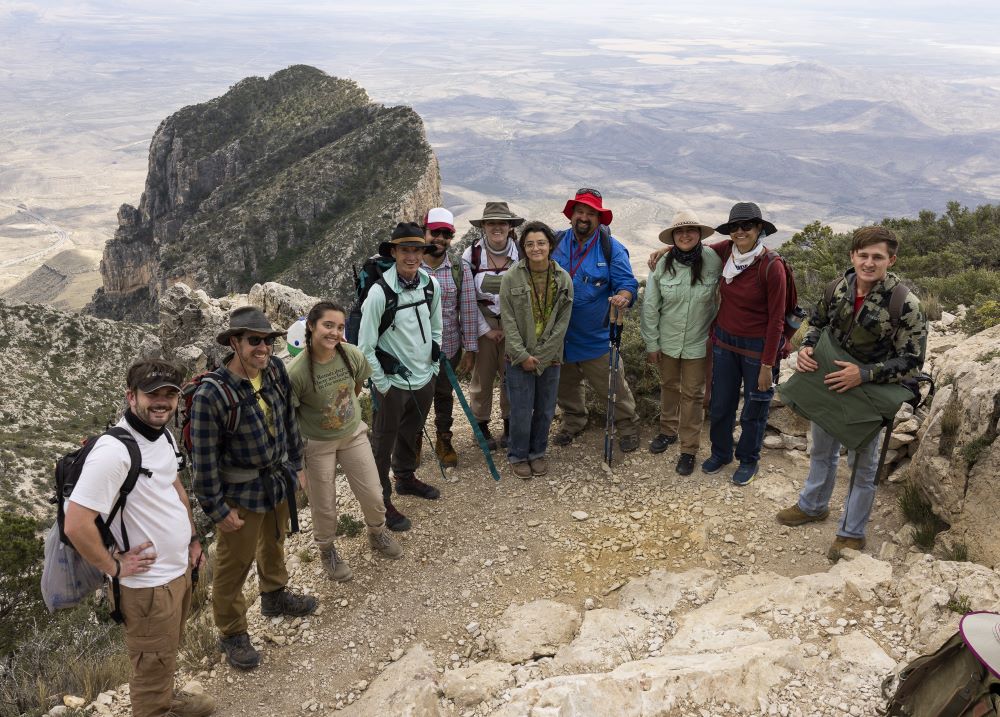
Plant biology research is incredibly diverse starting at soil and microbial interactions, to whole plant systems and genomes, to how the molecules and genes interact, to gene manipulation, to ecosystems and evolution. Our faculty's research issues address climate change, RNA mediated processes to understand genetic and biochemical processes, species relationships to reconstruct evolution genomics, transgenic crops that can combat abiotic stress, theoretical and mathematical models for water relations and ecology, the role of fire in arid lands, and how soil pollution affects environment and plants. This diverse area of plant biology has our graduate students working across Texas, the United States, chihuahua desert, Antarctica, Herbariums, as well as many international collaborations
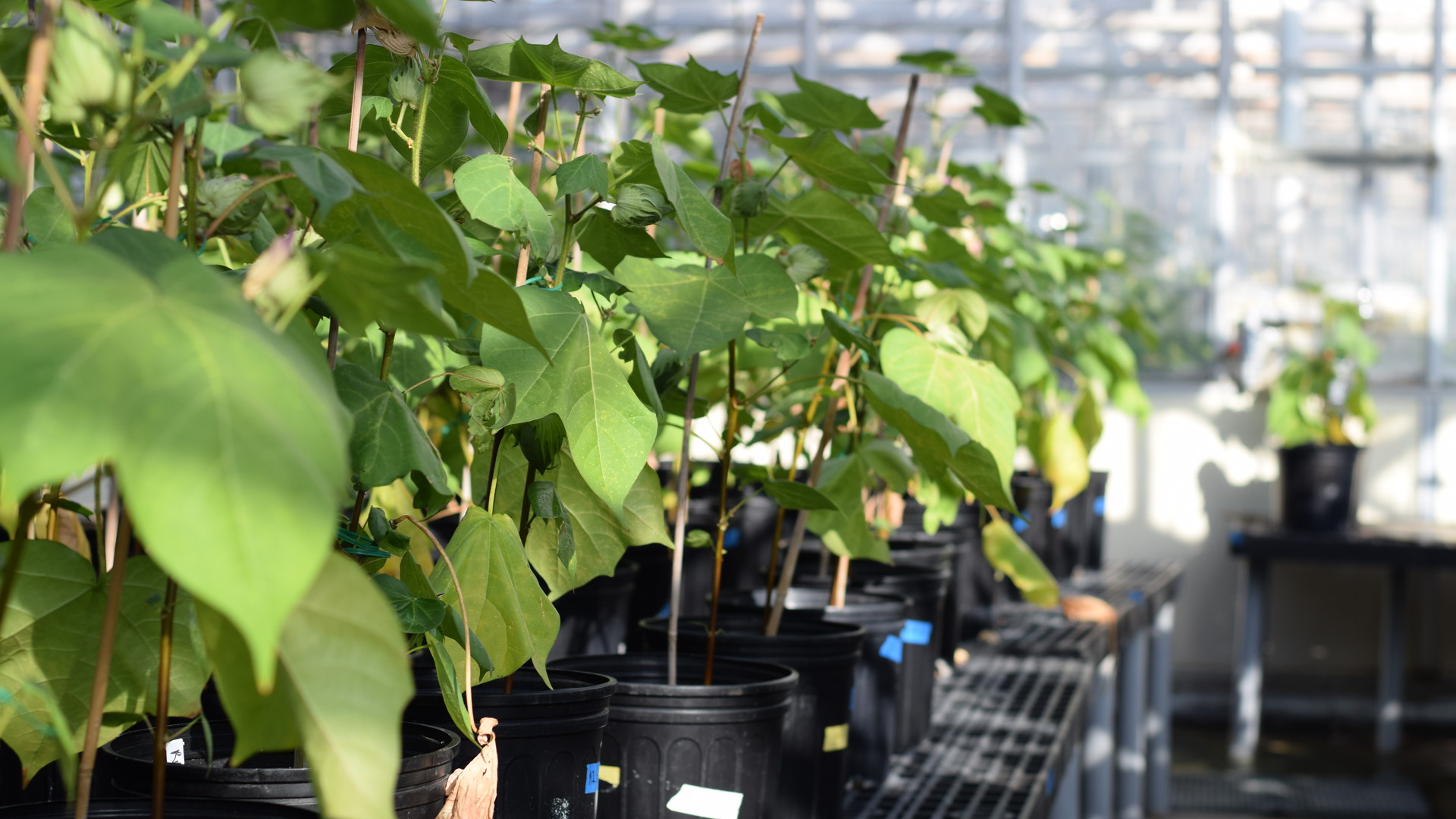
TTU Biology offers introductory plant courses for both majors and non-majors along with an introductory course for ecology and environmental problems. As your interest develops in plants there are many course offerings that explore principles of plant biology, evolutionary advances in plants, plant molecular biology, as well as the opportunities to do undergraduate research with professors and graduate students. Some courses have field trips to explore the concepts being learned or use our plant biology resources like the herbarium and greenhouse. Our undergraduate advisors can help select the best plant courses for your interest or research experience.
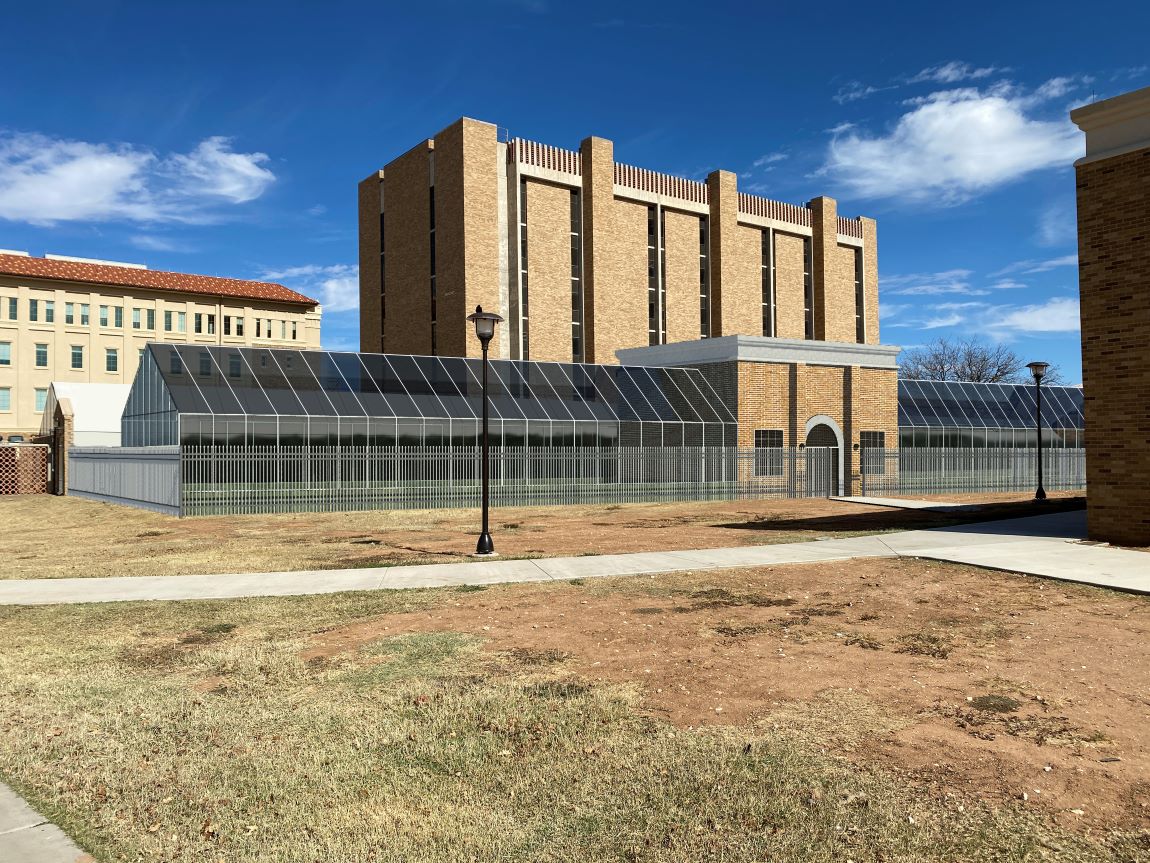
THE BIOLOGY GREENHOUSE
Inside you can find a diverse array of plants from row crops to grasses, grapevines, strawberries, and more surprises like the sansevieria collection from Grover Murray. While the current greenhouse opened its doors in 1987, current renovations and new designs are underway to enhance our controlled environment research space. Many graduate student projects are found inside along with a few class labs that enhance our teaching endeavors. Come step inside to see what the plant kingdom will showcase as our new research space is being built, starting in 2024! Read more about the Biology Greenhouse .
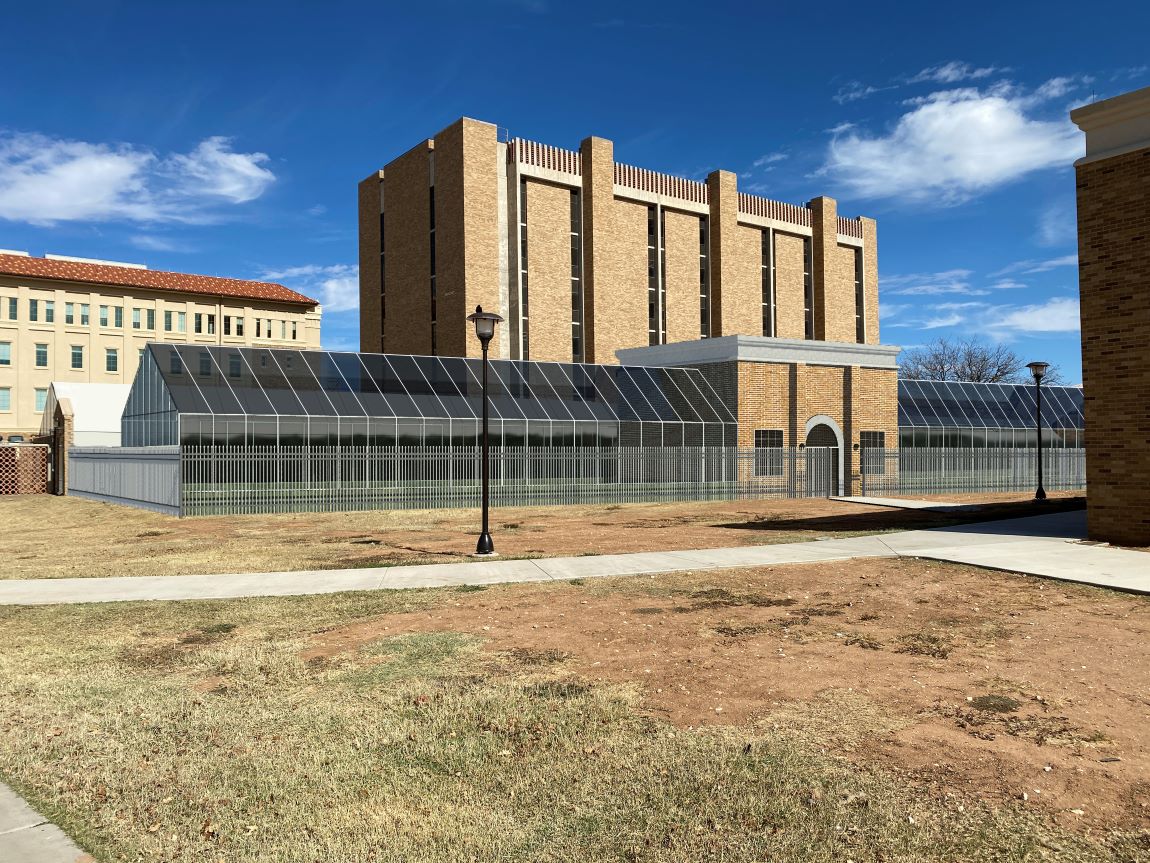
E.L. REED HERBARIUM at TTU
The E.L. Reed Herbarium at Texas Tech University houses over 25,000 dried, pressed plant specimens with collections focused on West Texas, the Southern Plains, and Southwest United States. The Herbarium, originally founded in 1925, is now located on the 7th floor roof of the Biology building and is the official plant repository for the Guadalupe Mountains National Park. Students working in the herbarium get experience with specimen curation, digitization, and plant systematics. Herbarium specimens are featured in teaching and research because they can function as a botanical time machine - providing insight on how the climate impacted plant genetics and physiology decades ago. Read more about the E.L. Reed Herbarium at TTU .
Contact TTU
An official website of the United States government
Here's how you know
Official websites use .gov A .gov website belongs to an official government organization in the United States.
Secure .gov websites use HTTPS. A lock ( Lock Locked padlock ) or https:// means you've safely connected to the .gov website. Share sensitive information only on official, secure websites.

- Search Awards
- Recent Awards
- Presidential and Honorary Awards
- About Awards
- How to Manage Your Award
- Grant General Conditions
- Cooperative Agreement Conditions
- Special Conditions
- Federal Demonstration Partnership
- Policy Office Website

Please report errors in award information by writing to: [email protected] .
- Open access
- Published: 03 June 2024
Screening and functional verification of drought resistance-related genes in castor bean seeds
- Zhiyan Wang 1 na1 ,
- Rui Luo 1 na1 ,
- Xiaotian Liang 2 , 3 ,
- Huibo Zhao 4 ,
- Yong Zhao 5 ,
- Mingda Yin 1 ,
- Yanpeng Wen 1 ,
- Xuemei Hu 1 &
- Fenglan Huang 6 , 7
BMC Plant Biology volume 24 , Article number: 493 ( 2024 ) Cite this article
14 Accesses
Metrics details
Drought is one of the natural stresses that greatly impact plants. Castor bean ( Ricinus communis L.) is an oil crop with high economic value. Drought is one of the factors limiting castor bean growth. The drought resistance mechanisms of castor bean have become a research focus. In this study, we used castor germinating embryos as experimental materials, and screened genes related to drought resistance through physiological measurements, proteomics and metabolomics joint analysis; castor drought-related genes were subjected to transient silencing expression analysis in castor leaves to validate their drought-resistant functions, and heterologous overexpression and backward complementary expression in Arabidopsis thaliana, and analysed the mechanism of the genes' response to the participation of Arabidopsis thaliana in drought-resistance.
Three drought tolerance-related genes, RcECP 63 , RcDDX 31 and RcA/HD1 , were obtained by screening and analysis, and transient silencing of expression in castor leaves further verified that these three genes corresponded to drought stress, and heterologous overexpression and back-complementary expression of the three genes in Arabidopsis thaliana revealed that the function of these three genes in drought stress response.
In this study, three drought tolerance related genes, RcECP 63 , RcDDX 31 and RcA/HD1 , were screened and analysed for gene function, which were found to be responsive to drought stress and to function in drought stress, laying the foundation for the study of drought tolerance mechanism in castor bean.
Peer Review reports
Introduction
Plant growth and development are mainly affected by many abiotic stresses such as salinity stress, drought stress, cold stress, etc., of which the impact of drought stress on plant growth is particularly prominent, in the process of plant growth and development is vulnerable to salinity, wet flooding, high temperature, low temperature, heavy metal ions and drought and other abiotic environmental stresses, which will reduce the yield of the crop, which drought caused by the most reduction in crop yields [ 1 , 2 , 3 ], therefore, the study of the drought tolerance of the plant and the cultivation of drought-resistant plant varieties in order to cope with the environment is more necessary.
Plant response to abiotic stresses such as drought is achieved through the regulation of gene expression. Plant responses to drought stress signals include both physiological responses at the cellular level and changes in gene expression. At the cellular level, the recognition, transduction and response to intercellular messengers through receptor cells will ultimately regulate the expression and metabolism of specific genes to improve drought resistance in plants [ 4 , 5 ]. In terms of gene expression, drought-resistant gene expression is altered by signal transduction after drought stress in plants thereby increasing drought resistance.
Castor bean ( Ricinus communis L.) is an annual or perennial herbaceous plant species belonging to the genus Ricinus in the family Euphorbiaceae [ 6 , 7 ]. It is an oil crop with high economic and use value [ 8 ]. Castor oil has many application values, and it is hailed as a renewable “petroleum resource” as the only vegetable oil that can replace petroleum. Therefore, it is of great significance to study castor bean.
In this study, we screened out the genes involved in drought stress regulation in castor bean embryos at the germination stage by using differential proteomics, comparative metabolomics, and reverse transcription–quantitative PCR (RT-qPCR) analysis. We overexpressed and complementarily expressed these genes into Arabidopsis thaliana and verified the functions of these genes through the analysis of gene expression levels, measurement of relevant physiological indicators, and phenotype analysis of T3 generation homozygous A. thaliana , aiming to lay a theoretical basis for determining the drought resistance mechanisms of castor bean.
Results and analysis
Physiological studies on the response of castor embryos to drought stress during germination, measurement of catalase (cat) activity.
The results are shown in Fig. 1 , with increasing processing time, water treatment shows a downward trend, 15% PEG treatment showed an increasing trend.
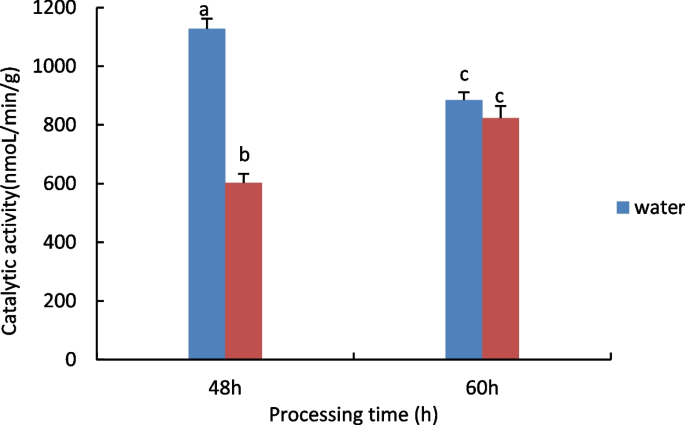
Results of CAT activity assay of castor embryos with different treatments. Note: Different lower case letters represent significant differences ( p < 0.05)
Measurement of Superoxide dismutase (SOD) activity
The results are shown in Fig. 2 , no significant difference in SOD activity between the 48 h water treatment and the 15% PEG 6000 treatment, when treated with stress for 60 h, compared to control, the SOD activity of castor seed embryos showed an increasing trend under 15% PEG treatment.
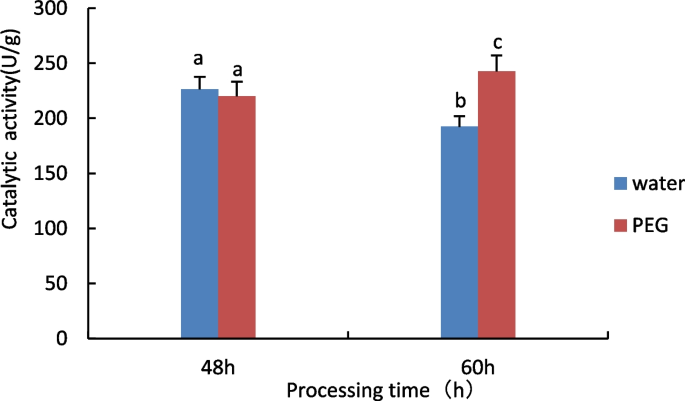
Results of SOD activity assay of castor embryos with different treatments. Note: Different lower case letters represent significant differences ( p < 0.05)
Measurement of Peroxidase (POD) activity
The results are shown in Fig. 3 , with increasing treatment time POD activity increased significantly in both the treated and control groups, and in the comparison of different treatments at the same time, the activity of POD in the control group was significantly higher than that in the treated group.
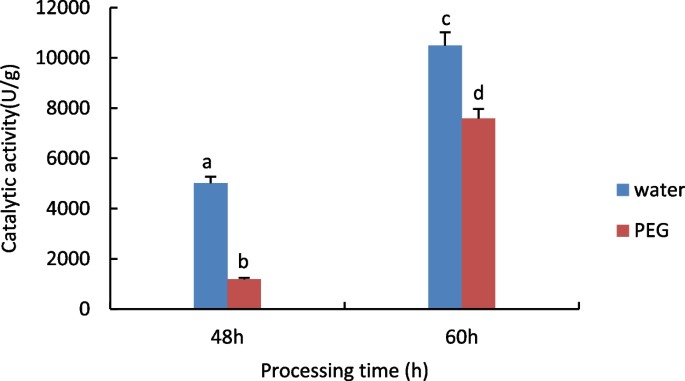
Results of POD activity assay of castor embryos with different treatments. Note: Different lower case letters represent significant differences ( p < 0.05)
Measurement of Glutathione S-transferase (GST) activity
The results are shown in Fig. 4 , with increasing processing time, GST activity was significantly reduced in both the control and treated groups, the treated group had higher GST activity than the control group.
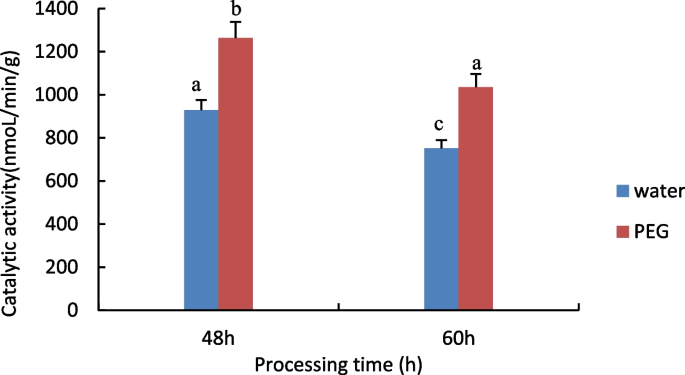
Results of GST activity assay of castor embryos with different treatments. Note: Different lower case letters represent significant differences ( p < 0.05)
Measurement of Total antioxidant capacity (T-AOC) capacity
The results are shown in Fig. 5 , compared to control, with increasing processing time, significant decrease in T-AOC activity of seed embryos, significant increase in T-AOC activity in the control group.
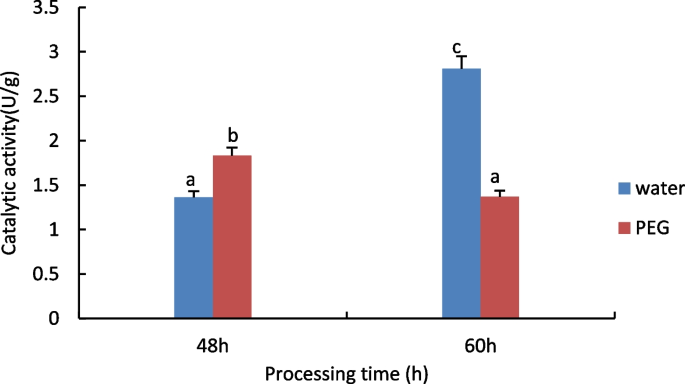
Results of T-AOC activity assay of castor embryos with different treatments. Note: Different lower case letters represent significant differences ( p < 0.05)
Measurement of Malondialdehyde (MDA) activity
The results are shown in Fig. 6 , compared to control, with increasing processing time, increase in MDA levels, while with increasing stress time, MDA content decreased in both water and 15% PEG 6000 treatments.
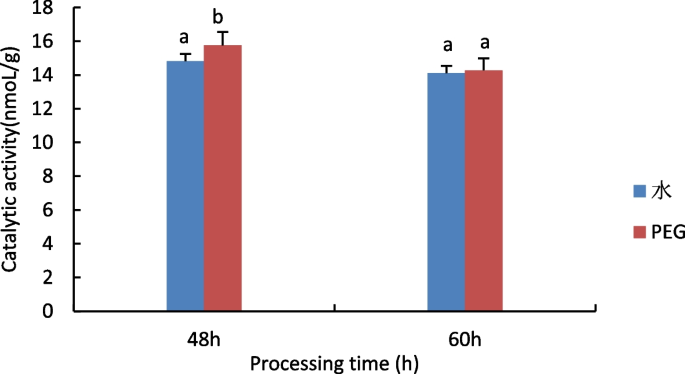
Results of MDA activity assay of castor embryos with different treatments. Note: Different lower case letters represent significant differences ( p < 0.05)
Measurement of Hydrogen peroxide (H2O2) activity
The results are shown in Fig. 7 , the level of hydrogen peroxide in both the treatment and control groups increased significantly with time, compared to the control group, The water treatment was significantly higher than the PEG treatment in the amount of hydrogen peroxide for the same treatment time.
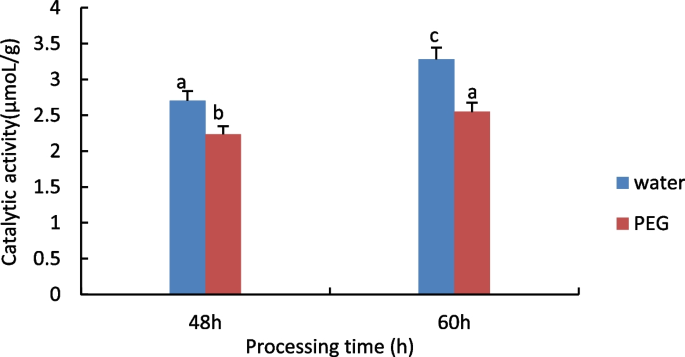
Results of H 2 O 2 activity assay of castor embryos with different treatments. Note: Different lower case letters represent significant differences ( p < 0.05)
Measurement of Proline (Pro) activity
The results are shown in Fig. 8 , compared to control, Pro content in embryos of all samples under PEG stress increased, Pro content was reduced in both water and PEG-treated castor embryos.The accumulation of Pro plays a decisive role in the resistance of the plant body, and Pro prevents changes in plasma membrane permeability and has a protective effect on the integrity of the plasma membrane, and the results of this experiment suggest that Pro responds to drought stress by exerting an osmoregulatory effect.
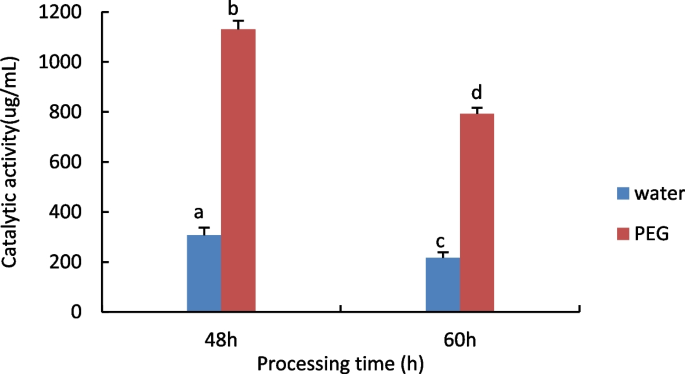
Results of Pro activity assay of castor embryos with different treatments. Note: Different lower case letters represent significant differences ( p < 0.05)
Determination of hydroxyl radical scavenging capacity
The results are shown in Fig. 9 , hydroxyl radical scavenging capacity decreases with time under water treatment conditions, while rising under 15% PEG treatment.
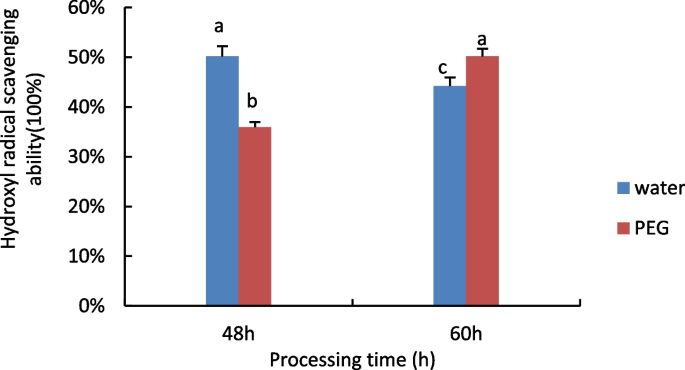
The transient silencing expression analysis of castor leaves revealed that three genes, RcECP 63 , RcDDX 31 and RcA/HD1 , were silenced, resulting in more severe surface wilting of castor leaves under drought stress conditions compared with the control; the expression of the three genes was significantly down-regulated after silencing; a significant increase in MDA content was found in the determination of physiological indicators, while the content of Pro, hydroxyl radical scavenging capacity and T-AOC showed a decreasing trend, indicating that the three selected genes have functions in the response to leaf involvement in drought stress, and it is hypothesized that these three genes are also involved in drought stress during the sprouting stage of castor.
Differential proteomic and widely targeted metabolomic analyses of castor bean embryos at the germination stage
Differential proteomics, differentially expressed protein analysis.
The iTRAQ technique was used to perform proteomic analysis on castor embryo samples with different treatments. The total number of identified peptides was 13,971; the number of identified unique peptides was 9,592; and the number of identified proteins was 2,101. The Venn diagram and volcano plots of differentially expressed proteins are shown in Figs. 10 and 11 . In the comparison group P48_VS_P60, there were 115 and 15 upregulated and downregulated proteins, respectively. In the comparison group S48_VS_P48, there were 18 and 106 upregulated and downregulated proteins, respectively. In the comparison group S60_VS_P60, there were 26 and 33 upregulated and downregulated proteins, respectively. In the comparison group S48_VS_S60, there were 59 and 29 upregulated and downregulated proteins, respectively.
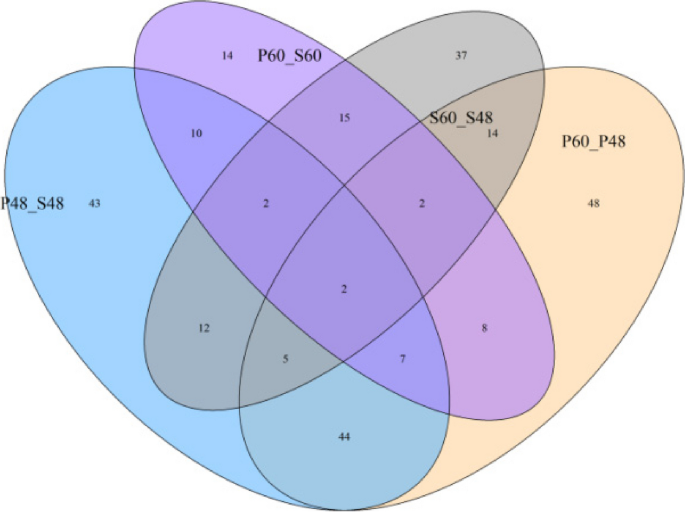
Venn diagram of differentially expressed proteins
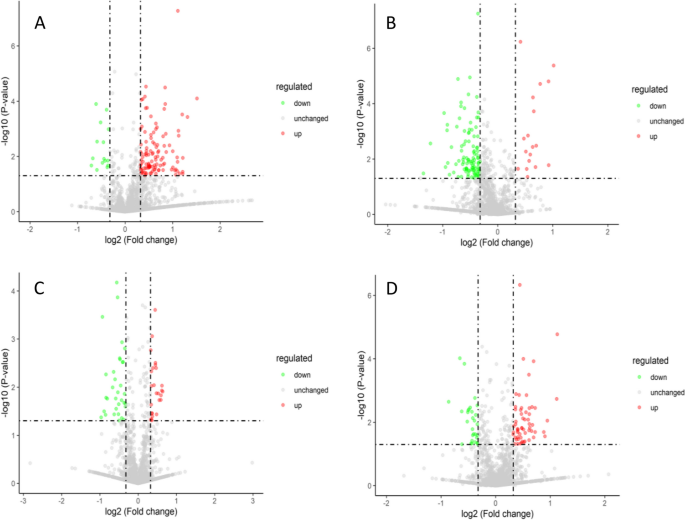
Volcano plots of differentially expressed proteins. Note: A-D show the differentially expressed proteins screened out in the four comparison groups: P48_VS_P60, S48_VS_P48, S60_VS_P60, and S48_VS_S60, respectively. The green dots represent the differentially expressed proteins with downregulated expression, the red dots represent the differentially expressed proteins with upregulated expression, and the gray dots represent the detectable proteins with no significant changes in expression
Hierarchical clustering results of differentially expressed proteins
Figure 12 shows the results of hierarchical clustering analysis of the selected differentially expressed proteins. As the treatment concentration and treatment duration increased, the number of upregulated proteins significantly increased. We speculate that the longer drought stress elicited a more sensitive response of castor bean embryos to the external environment.
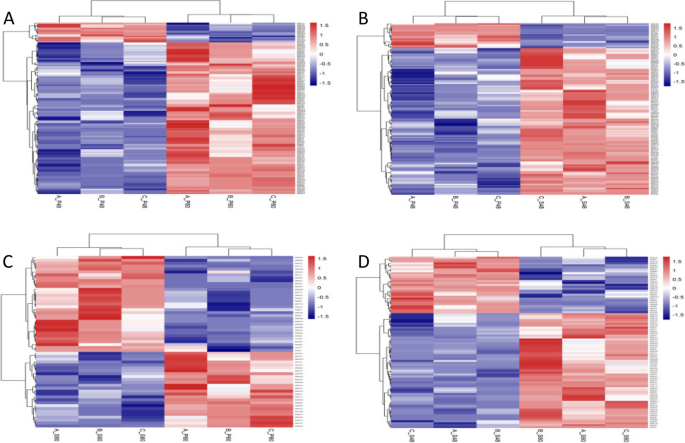
Hierarchical clustering analysis of differentially expressed proteins. Note: A-D show the results of hierarchical clustering analysis of the differentially expressed proteins in the four comparison groups P48_VS_P60, S48_VS_P48, S60_VS_P60, and S48_VS_S60, respectively
Gene Ontology functional annotation analysis of differentially expressed proteins
To further study the biological processes involving differentially expressed proteins, Gene Ontology functional enrichment analysis was performed on the differentially expressed proteins. The results in Fig. 13 indicate that the differentially expressed proteins participated in the whole life cycle through biological processes, cellular components, and molecular functions. As the drought stress is prolonged, the external environment influences the germination of castor bean seeds by changing not only the material and energy transport but also the biosynthesis and metabolism of substances.
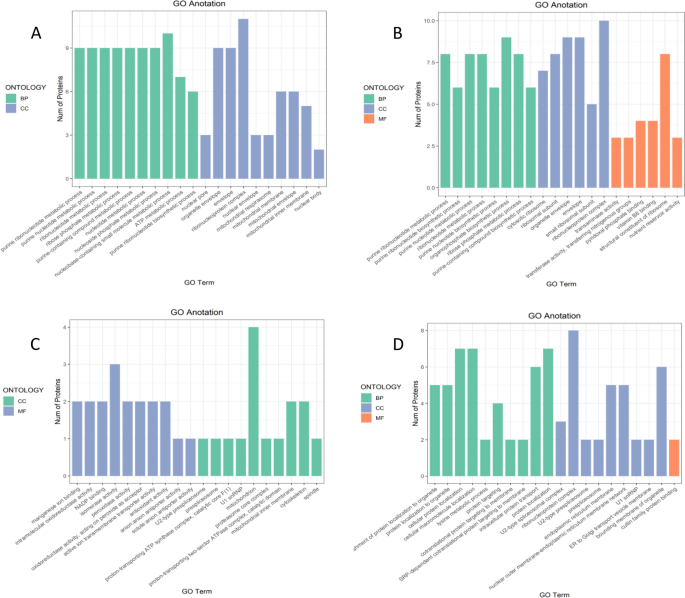
Gene Ontology functional annotation analysis of differentially expressed proteins. Note: A-D show the annotation results of differentially expressed proteins in the four comparison groups P48_VS_P60, S48_VS_P48, S60_VS_P60, and S48_VS_S60, respectively
Widely targeted metabolomics
Volcano plots of differentially expressed metabolites.
To analyze the fold changes and intergroup differences in the metabolite levels in the samples, the differentially expressed metabolites of the four groups (P48_VS_P60, S48_VS_P48, S60_VS_P60, and S48_VS_S60) of samples were analyzed by volcano plots. The results are shown in Fig. 14 . The variable importance in projection (VIP) values of all metabolites were greater than 1, which indicates that the differential expression of metabolites was significant and that the screening of differentially expressed metabolites was reliable. Moreover, as the PEG 6000 concentration and the stress duration increased, the differentially expressed metabolites of the castor bean embryos changed more obviously, which indicates that abiotic stress had a more significant regulatory effect on the metabolites.
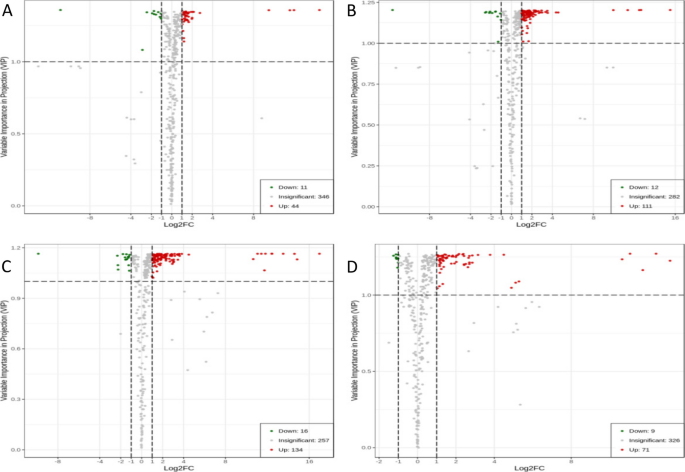
Volcano plots of differentially expressed metabolites. Note: A-D show the volcano plots of the differentially expressed metabolites in the four comparison groups P48_VS_P60, S48_VS_P48, S60_VS_P60, and S48_VS_S60, respectively. VIP represents the significance of the difference in a given metabolite between groups. Log 2 FC represents the fold change of the differentially expressed metabolite. The green dots represent the downregulated metabolites, the red dots represent the upregulated metabolites, and the gray dots represent the detectable metabolites with no significant changes in concentration
Differentially expressed metabolite screening results
A total of 408 differentially expressed metabolites were screened out in the four groups (P48_VS_P60, S48_VS_P48, S60_VS_P60, and S48_VS_S60) of samples. Among them, 123 differentially expressed metabolites were identified in P48_VS_S48, 55 were identified in P48_VS_P60, 80 were identified in S48_VS_S60, and 150 were identified in P60_VS_S60. The Venn diagram of differentially expressed metabolites in the four groups is shown in Fig. 15 .
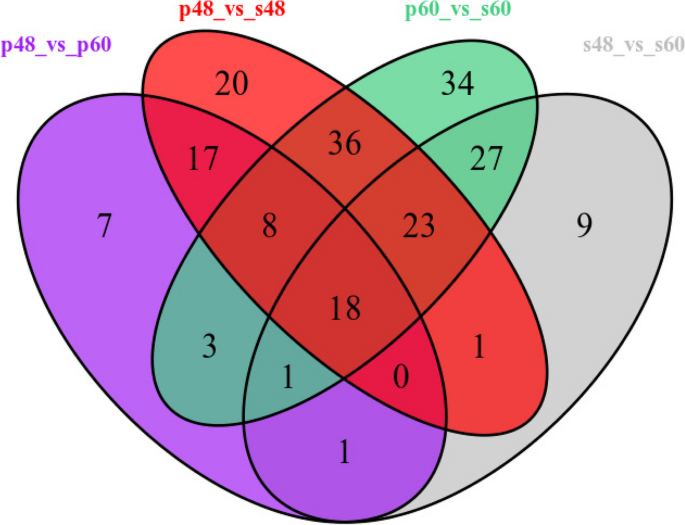
Venn diagram of differentially expressed metabolites in groups P48_VS_P60, S48_VS_P48, S60_VS_P60, and S48_VS_S60
KEGG functional annotation analysis of differentially expressed metabolites
The differentially expressed metabolites screened out in the four comparison groups (P48_VS_P60, S48_VS_P48, S60_VS_P60, and S48_VS_S60) were subjected to functional annotation analysis of KEGG metabolic pathways. The results in Figs. 16 , 17 , 18 and 19 show that the highest proportion of differentially expressed metabolites in group P48_VS_P60 were annotated in the metabolic pathways, biosynthesis of secondary metabolites, phenylpropanoid biosynthesis, and glycerolipid metabolism; that the highest proportion of differentially expressed metabolites in group P48_VS_S48 were annotated in the metabolic pathways and biosynthesis of secondary metabolites; that the highest proportion of differentially expressed metabolites in group P60_VS_S60 were annotated in the metabolic pathways and biosynthesis of secondary metabolites; and that the highest proportion of differentially expressed metabolites in group S48_VS_S60 were annotated in the biosynthesis of secondary metabolites, flavonoid biosynthesis, and phenylpropanoid biosynthesis.
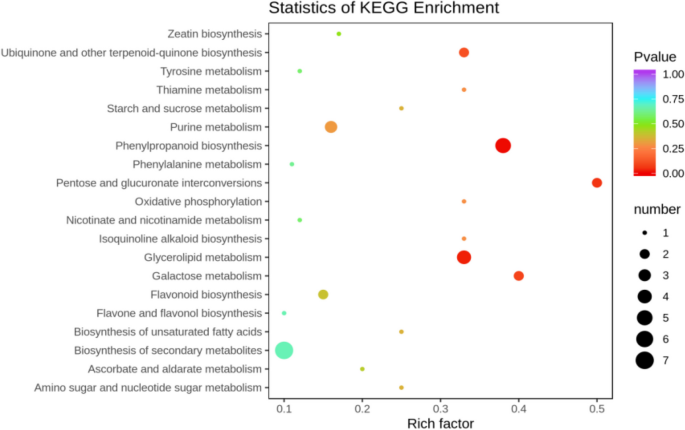
KEGG enrichment analysis of the differentially expressed metabolites in group P48_VS_P60
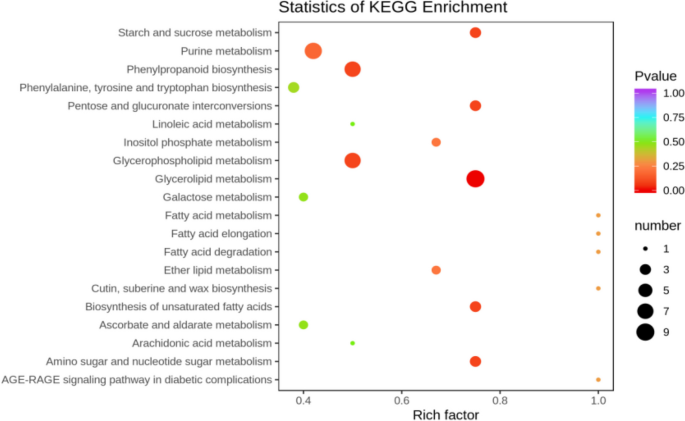
KEGG enrichment analysis of the differentially expressed metabolites in group P48_VS_S48
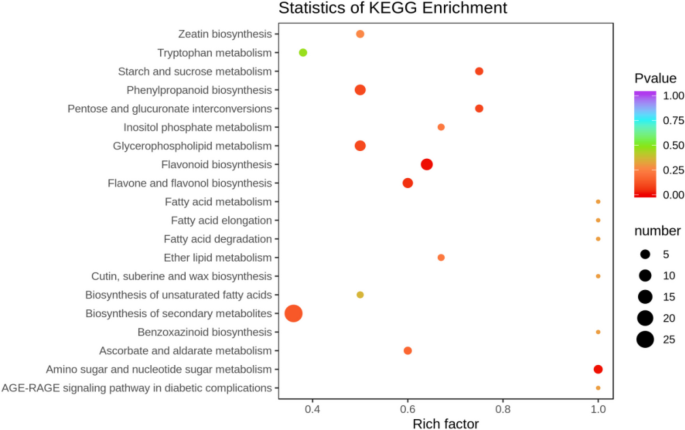
KEGG enrichment analysis of the differentially expressed metabolites in group P60_VS_S60
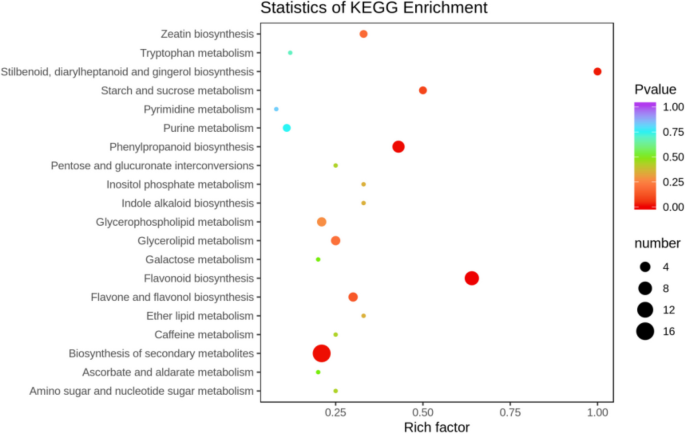
KEGG enrichment analysis of the differentially expressed metabolites in group S48_VS_S60
Results of combined differential proteomics and comparative metabolomics
To further determine the effects of different drought stress treatments on the metabolites in the germination stage of castor bean plants and the metabolic pathways in response to drought stress treatments, the differential proteomic analysis of groups P48_VS_P60, P48_VS_S48, P60_VS_S60, and S48_VS_S60 were combined with the results of comparative metabolomic analysis. We used the KEGG metabolic pathways to map the common metabolic pathways of the differentially expressed proteins and differentially expressed metabolites.
Results of combined proteomic and metabolomic analysis of group P48_VS_P60
Figure 20 shows the results of combined analysis of the proteomic and metabolomic data of group P48_VS_P60. The ascorbate and aldarate metabolism and purine metabolism were the common metabolic pathways of the differentially expressed proteins and differentially expressed metabolites of group P48_VS_P60.
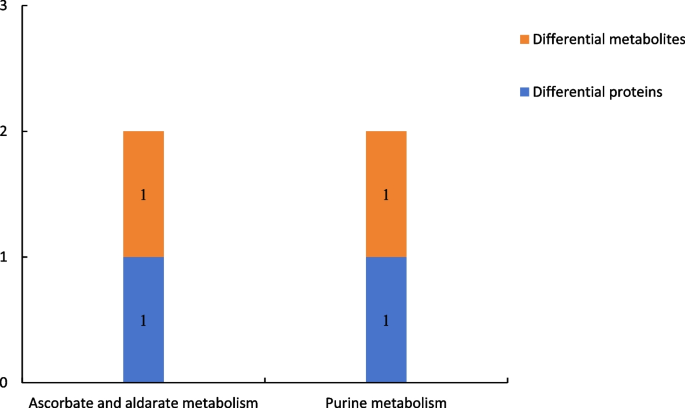
Common metabolic pathways of the differentially expressed proteins and differentially expressed metabolites in group P48_VS_P60. Note: The abscissa represents the metabolic pathways, and the ordinate represents the number of differentially expressed proteins and differentially expressed metabolites simultaneously involved in each metabolic pathway
Results of combined proteomic and metabolomic analysis of group P48_VS_S48
Figure 21 shows the results of combined analysis of the proteomic and metabolomic data of group P48_VS_S48. The common metabolic pathways of the differentially expressed proteins and differentially expressed metabolites of group P48_VS_S48 included biosynthesis of amino acids, tyrosine metabolism, and 2-oxycarboxylic acid metabolism.
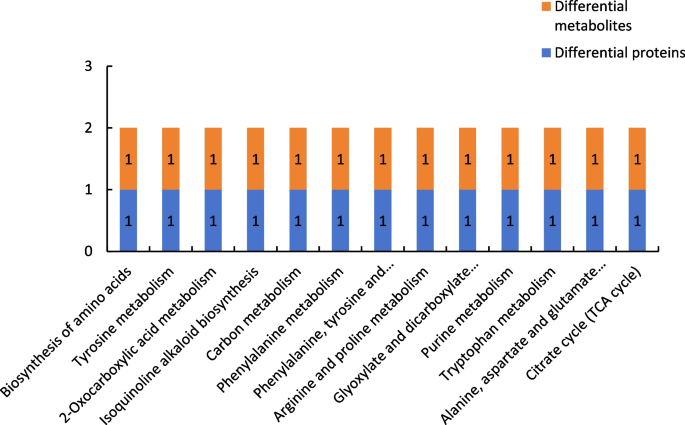
Common metabolic pathways for the differentially expressed proteins and differentially expressed metabolites of group P48_VS_S48. Note: The abscissa represents the metabolic pathways, and the ordinate represents the number of differentially expressed proteins and differentially expressed metabolites simultaneously involved in each metabolic pathway
Results of combined proteomic and metabolomic analysis of group P60_VS_S60
Figure 22 shows the results of combined analysis of the proteomic and metabolomic data of group P60_VS_S60. The common metabolic pathways of the differentially expressed proteins and differentially expressed metabolites of group P60_VS_S60 included carbon metabolism and stilbenoid, diarylheptanoid, and gingerol biosynthesis.
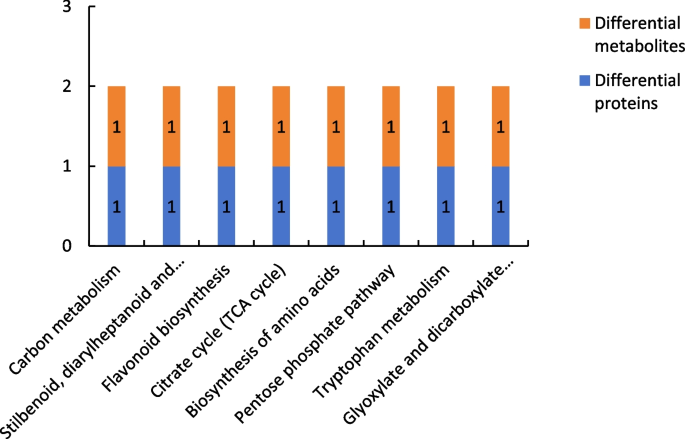
Common metabolic pathways for the differentially expressed proteins and differentially expressed metabolites of group P60_VS_S60. Note: The abscissa represents the metabolic pathways, and the ordinate represents the number of differentially expressed proteins and differentially expressed metabolites simultaneously involved in each metabolic pathway
Results of combined proteomic and metabolomic analysis of group S48_VS_S60
The results of combined analysis of the proteomic and metabolomic data of group S48_VS_S60 showed that there were three pathways for differentially expressed proteins in water-treated plants at different times, namely, protein processing in the endoplasmic reticulum, protein export, and ubiquitin-mediated proteolysis. The differentially expressed metabolites of group S48_VS_S60 were mainly involved in the biosynthesis and metabolism of amino acids. The proteomic and metabolomic data did not share any common metabolic pathways.
RT-qPCR analysis of drought resistance-related genes
RT-qPCR analysis was performed on the genes ( RcECP63 , RcDDX31 , RcA/HD1 , RcRANGAP1 , RcITP , RcGST1 , RcLEAD7 , RcVTVS13 ) encoding eight differentially expressed proteins (B9SRL2, B9SBJ5, B9SHP2, B9S424, B9REZ2, B9RCA3, B9RV15, B9RCA3 B9SVJ2) that might be involved in the drought stress response to study their expression patterns at the transcriptional level. The results are shown in Fig. 23 .
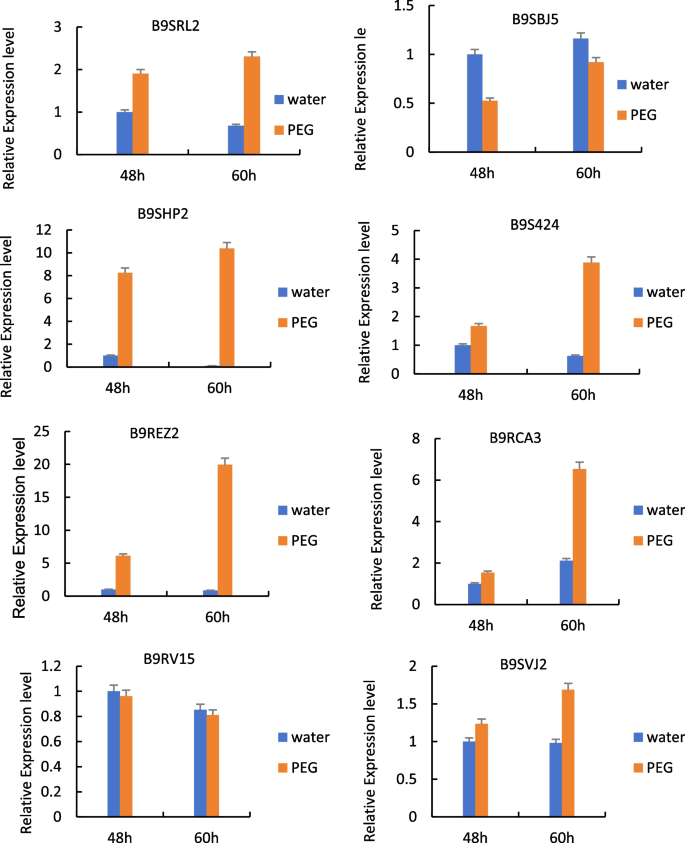
RT-qPCR detection results of the genes encoding some differentially expressed proteins in castor plants in response to drought stress. Note: Different lowercase letters represent significant differences ( P <0.05)
Expression analysis of transient silencing of differential genes in castor bean ( Ricinus communis L.)
Results of phenotyping of leaves.
Before the stress treatment, castor leaves had good growth and were in a more consistent state; when 15% PEG was treated for 24 h, it could be seen that there was obvious wilting of the leaves and obvious curling occurred at the edges of the leaves; when 15% PEG was treated for 48 h, the loss of water in the leaves was more serious and the curling phenomenon was more obvious, which indicated that at this point in time, after the silencing of the studied genes, RcECP 63 , RcDDX 31 , and RcA/HD1 , the leaves were more intolerant of the drought stress.The result is shown in the Fig. 24 .
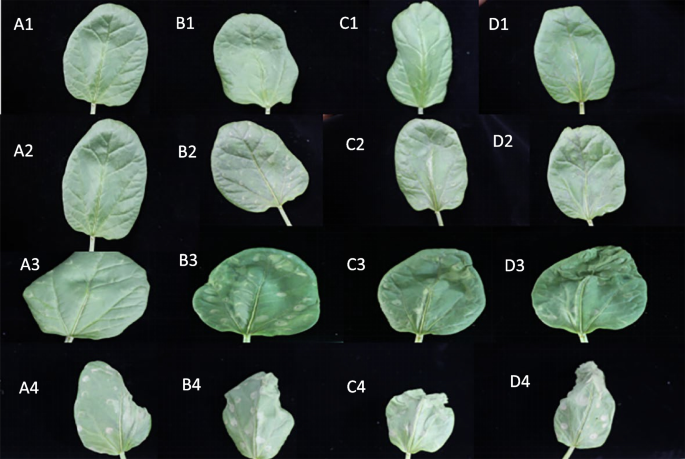
Phenotypic changes of castor bean leaves after transient infection. Note: A1-A4 are the leaf states of wild-type castor leaves treated with 15% PEG 6000 for 0 h, 0 h, 24 h and 48 h respectively; B1-B4 are the leaf states treated with 15% PEG 6000 for 0 h, 24 h and 48 h before and after PTRV-RcECP 63 bacterial solution; C1-C4 are the leaf states treated with 15% PEG 6000 for 0 h, 24 h and 48 h before impregnation with PTRV-RcDDX 31 and after injection of PTRV-RcDDX 31 bacterial solution; D1-D4 are the leaf states treated with 15% PEG 6000 for 0 h, 24 h and 48 h before impregnation with PTRV-RcA/HD1 and after injection of PTRV-RcA/HD1 bacterial solution
Results of RT-qPCR analysis of differentially expressed genes in leaves
As can be seen from Fig. 25 , when castor plants were subjected to drought stress, the expression of the RcECP 63 gene in wild-type castor leaves was significantly up-regulated with the increase of drought stress time; whereas the expression of the gene in castor leaves injected with recombinant PTRV- RcECP 63 bacterial broth under the same treatment conditions all showed a down-regulation trend, which was even more pronounced with the prolongation of time, indicating that the gene was silenced.
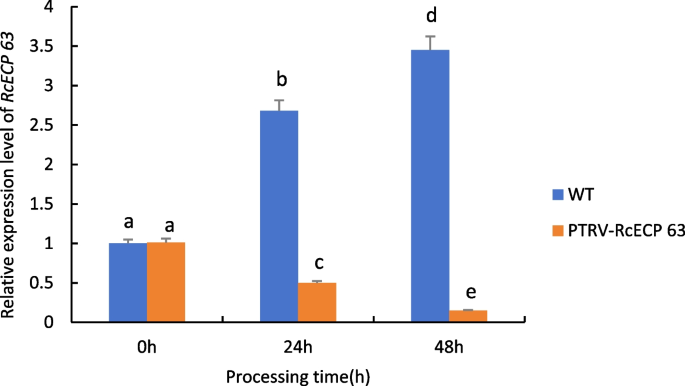
Expression analysis of RcECP 63 gene transiently infected castor leaves under drought stress. Note: Different lowercase letters represent significant differences( P < 0.05)
As can be seen from Fig. 26 , when castor plants were subjected to drought stress, the expression of the RcDDX 31 gene in wild-type castor leaves showed a significant up-regulation trend with the increase of drought stress time; whereas the expression of the gene in castor leaves injected with recombinant PTRV- RcDDX 31 bacterial broth under the same treatment conditions showed a significant down-regulation trend, which was even more pronounced with the increase of time, indicating that the gene was silenced.
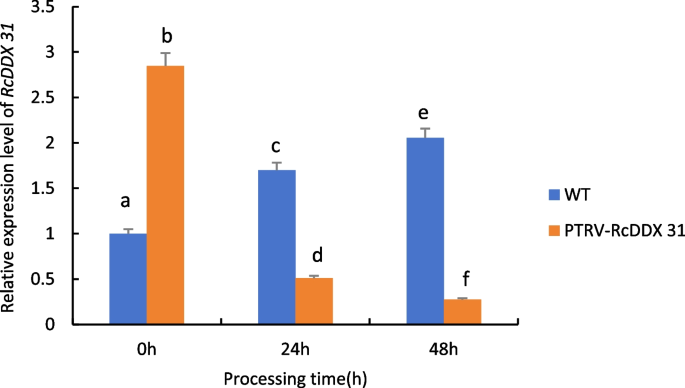
Expression analysis of RcDDX 31 gene transiently infected castor leaves under drought stress. Note: Different lowercase letters represent significant differences ( P < 0.05)
As can be seen from Fig. 27 , when castor plants were subjected to drought stress, the expression of the RcA/HD1 gene in wild-type castor leaves was significantly up-regulated with the increase of drought stress time; whereas the expression of the gene in castor leaves injected with recombinant PTRV- RcA/HD1 bacterial fluids under the same treatment conditions all showed a down-regulation trend, which was even more pronounced with the prolongation of time, indicating that the gene was silenced.
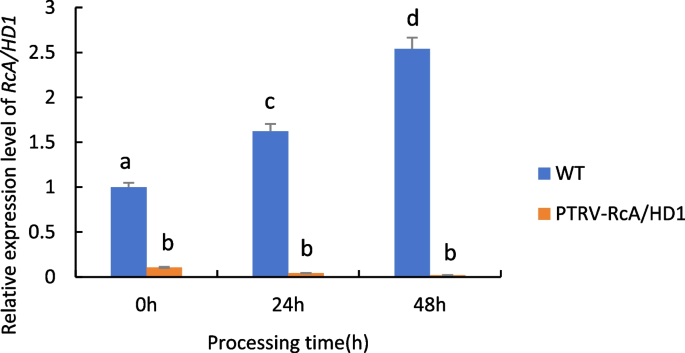
Expression analysis of RcA/HD1 gene transiently infected castor leaves under drought stress. Note: Different lowercase letters represent significant differences ( P < 0.05)
Combining the experimental results of the three sets of data, it can be seen that when the three selected genes were transiently silenced, the expression of the genes showed a significant down-regulation compared to the wild-type control, in which the expression of the RcA/HD1 gene was less and the decline was more pronounced, which further suggests that the three selected genes are associated with drought resistance in castor bean.
Functional analysis of differentially expressed genes in A. thaliana
Vector construction and identification of expression plants.
The specific primers were used to amplify the target genes RcECP 63 , RcDDX 31 and RcA/HD1 , respectively, and Nco I single enzyme digestion was performed on the pairs of heterologous expression vector pCAMBIA1305.2, and the results were all available for the next step of the experiment. The constructed vector plasmid was transformed into Agrobacterium GV3101 strain. The recombinant Agrobacterium sap was used to dip Arabidopsis thaliana (wild type and its mutants Col-0, atecp63 , atddx31-1 , atddx31-2 , ata/hd1 ) using the flower dipping method, and overexpression-resistant plants ECP 63-OE , DDX 31-OE , A/HD1-OE and back-expression-resistant plants ECP 63 -The transgenic plants were analysed at the molecular and biological levels to determine the gene functions.
PCR identification of overexpressing Arabidopsis plants and back-expressing Arabidopsis plants showed that seven overexpressing Arabidopsis ECP 63-OE , five overexpressing Arabidopsis DDX 31-OE , and five overexpressing Arabidopsis A/HD1-OE plants were obtained, as well as seven back-expressing Arabidopsis ECP 63-GR , seven back-expressing Arabidopsis DDX31-GR1 , and four back-expressing Arabidopsis A/HD1-GR . The results are shown in Figs. 28 , 29 and 30 .
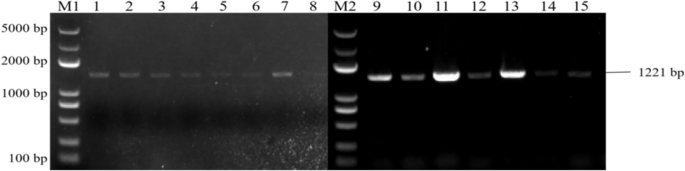
Results of PCR identification of the resistant Arabidopsis thaliana plants with overexpression and complementary expression of RcECP63 . Note: M1 and M2: DL 5000 Marker; 1-8: PCR identification results of the resistant complementary-expression A. thaliana plant ECP63-GR . 9-15: PCR identification results of the resistant overexpression A. thaliana plant ECP63-OE
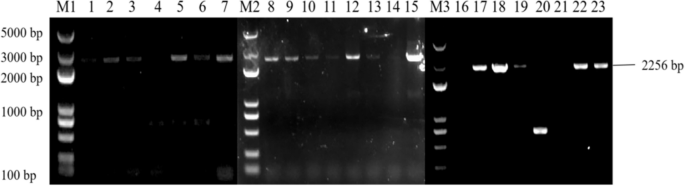
Results of PCR identification of the resistant Arabidopsis thaliana plants with overexpression and complementary expression of RcDDX31 . Note: M1, M2, and M3: DL 5000 Marker; 1-7: PCR identification results of the resistant complementary-expression A. thaliana plant DDX31-GR1 ; 8-15: PCR identification results of the resistant complementary-expression A. thaliana plant DDX31-GR2 ; 16-23: PCR identification results of the resistant overexpression A. thaliana plant DDX31-OE
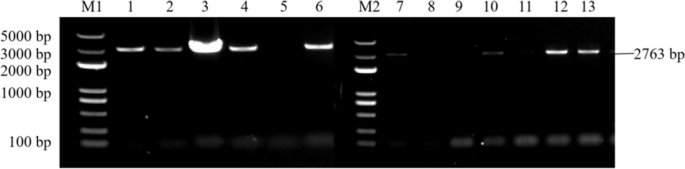
Results of PCR identification of the resistant Arabidopsis thaliana plants with overexpression and complementary expression of RcA/HD1 . Note: M1 and M2: DL 5000 Marker; 1-6: PCR identification results of the resistant complementary-expression A. thaliana plant A/HD1-GR ; 7-13: PCR identification of the resistant overexpression A. thaliana plant A/HD1-OE
RT-qPCR identification results of overexpression and complementary- expression transgenic A. thaliana plants
RNA was extracted from overexpressing and back-expressing Arabidopsis transgenic plants, and the samples were analysed by RT-qPCR using RNA reverse-transcribed cDNA as a template.
Figure 31 shows the RT-qPCR analysis of the samples with the reverse-transcribed cDNA of the overexpression plants as the template. Compared with the controls, the gene expression levels of the overexpression plants were significantly increased under drought stress. This indicates that due to changes in the environment, the transcripts of genes are significantly increased during the transcription process, which allows the plants to respond to drought stress.
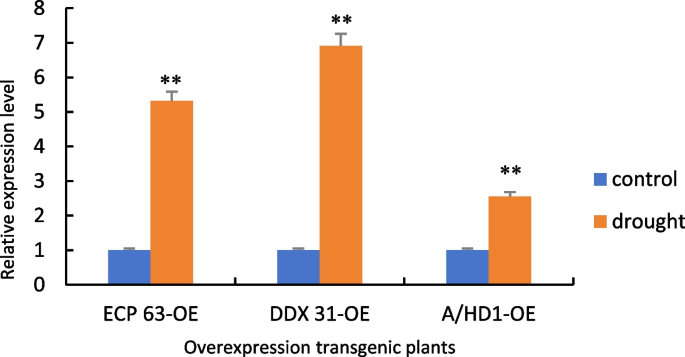
RT-qPCR results of positive overexpression Arabidopsis thaliana plants. Note: *: 0.01 < P ≤0.05, **: 0< P ≤0.01
Figure 32 shows the RT-qPCR analysis of the samples with the cDNA obtained from the reverse transcription of the complementary-expression plants as the template. Compared with the controls, the gene expression levels of the four types of plants with complementary expression of RcECP63 and RcDDX31 were significantly increased under drought stress, while the gene expression levels of the plants with complementary expression of RcA/HD1 were significantly reduced under drought stress. These results indicate that the plants with the complemented deletion mutant of RcA/HD1 had an insignificant response to drought stress.
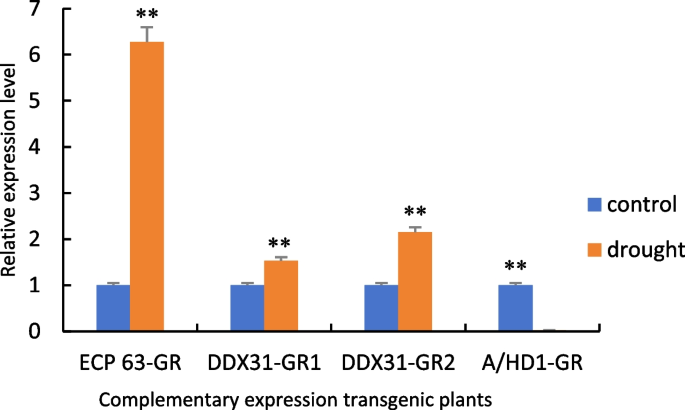
RT-qPCR results of positive complementary-expression Arabidopsis thaliana plants. Note: *: 0.01 < P ≤0.05, **: 0< P ≤0.01
Analysis of the tolerance of overexpression and complementary-expression A. thaliana plants to drought stress
Analysis of the response of the rcecp63 gene to drought stress.
We analyzed the effects of drought stress treatments with different mannitol concentrations on the growth and development of mutant, complementary-expression, wild-type, and overexpression A. thaliana (atecp63 , ECP63-GR , Col-0, and ECP63-OE ) and analyzed the germination rates, root lengths, and numbers of lateral roots of A. thaliana plants under drought stress treatments with different mannitol concentrations. The results are shown in Figs 33 and 34 . The plants without mannitol treatment grew normally. The drought stress significantly changed the morphology of A. thaliana . When A. thaliana plants were treated with 100 mmol/L mannitol, the overexpression A. thaliana plants showed significantly shorter root lengths and significantly more lateral roots than the controls. When A. thaliana plants were treated with 200 mmol/L mannitol, the number of lateral roots was still significantly increased. When A. thaliana plants were treated with 300 mmol/L mannitol, the number of lateral roots and the length of lateral roots were significantly downregulated, and the abaxial epidermis of the leaves of all four types of A. thaliana plants turned reddish-brown, especially in the mutant A. thaliana plants, and the A. thaliana plants suffered severe inhibition of their growth and development and severe damage.
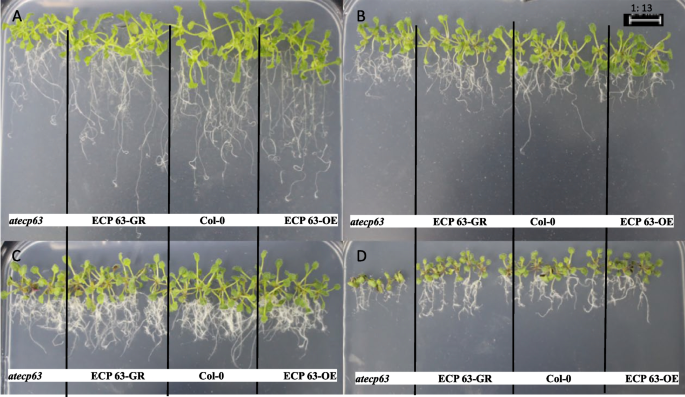
Effect of different concentrations of mannitol on atecp63 , ECP 63-GR , Col-0, and ECP 63-OE . Note: A-D represent the mannitol medium containing 0 mmol/L, 100 mmol/L, 200 mmol/L, and 300 mmol/L mannitol, respectively
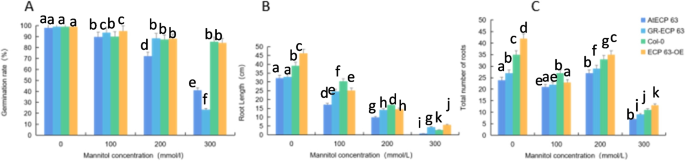
Germination rate, root length, and number of lateral roots of the four types of Arabidopsis thaliana plants by RcECP63 genotype. Note: Figure A shows the germination rate of A. thaliana under drought stress treatments with different mannitol concentrations; Figure B shows the root lengths of A. thaliana plants treated with different mannitol concentrations; Figure C shows the numbers of lateral roots of A. thaliana plants treated with different mannitol concentrations. Different lowercase letters represent significant differences ( P <0.05)
Analysis of the response of the RcDDX31 gene to drought stress
We analyzed the effects of drought stress treatments with different mannitol concentrations on the growth and development of mutant, complementary-expression, wild-type, and overexpression A. thaliana ( atddx31-1 , DDX31-GR1 , Col-0, and DDX31-OE ) and analyzed the germination rates, root lengths, and numbers of lateral roots of A. thaliana plants under drought stress treatments with different mannitol concentrations. The results are shown in Figs. 35 and 36 . A. thaliana grew normally without stress treatment, and the complementary-expression plant DDX31-GR1 had the longest root length. Drought stress changed the morphology of A. thaliana significantly. When A. thaliana plants were treated with 100 mmol/L mannitol, the root length was significantly shorter, and the number of lateral roots significantly increased compared with that of the controls. When A. thaliana plants were treated with 200 mmol/L mannitol, the number of lateral roots was significantly increased. When A. thaliana plants were treated with 300 mmol/L mannitol, the number of lateral roots was even less, and the length of lateral roots were even shorter, and the abaxial epidermis of the leaves of all four types of A. thaliana plants turned reddish-brown, which indicates that the tolerance of A. thaliana to mannitol was reduced at this concentration.
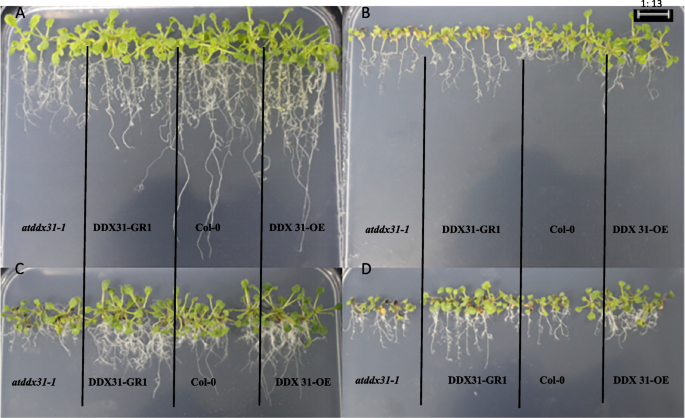
Effect of different mannitol concentration on atddx31-1 , DDX31-GR1 , Col-0, and DDX31-OE . Note: A-D represents the mannitol medium containing 0 mmol/L, 100 mmol/L, 200 mmol/L, and 300 mmol/L mannitol, respectively
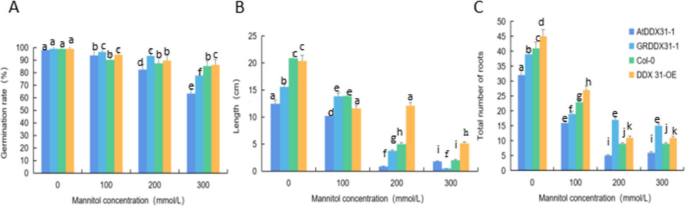
Analysis results of the germination rates, root lengths, and numbers of lateral roots of the four types of Arabidopsis thaliana corresponding to the RcDDX31 mutant atddx31-1 . Note: Figure A shows the germination rates of A. thaliana drought stress treatments with different mannitol concentrations. Figure B shows the root lengths of A. thaliana plants under drought stress treatments with different mannitol concentrations. Figure C shows the numbers of lateral roots of A. thaliana plants under drought stress treatments with different mannitol concentrations. Different lowercase letters represent significant differences ( P <0.05)
We analyzed the effects of drought stress treatments with different mannitol concentrations on the growth and development of mutant, complementary-expression, wild-type, and overexpression A. thaliana plants ( atddx31-2 , DDX31-GR2 , Col-0, and DDX31-OE ) and analyzed the germination rates, root lengths, and numbers of lateral roots of A. thaliana plants under drought stress treatments with different mannitol concentrations. The results are shown in Figs. 37 and 38 . The plants grew normally without stress treatment. Drought stress changed the morphology of A. thaliana significantly. When A. thaliana plants were treated with 100 mmol/L mannitol, the numbers of lateral roots in the mutant and complementary-expression plants were less increased, and a small number of leaves were chlorotic. When A. thaliana plants were treated with 200 mmol/L mannitol, the overall growth was better, and the number and length of lateral roots further increased, and the leaves of mutant and complementary-expression plants turned chlorotic or reddish-brown. When A. thaliana plants were treated with 300 mmol/L mannitol, the number and length of lateral roots were less than when they were treated with 100 mmol/L and 200 mmol/L mannitol, and all leaves turned reddish-brown, but the overexpression and complementary-expression plants had more and longer lateral roots than the wild-type and mutant plants, which indicates that the response of this gene to drought stress was mainly manifested in the number and length of lateral roots.
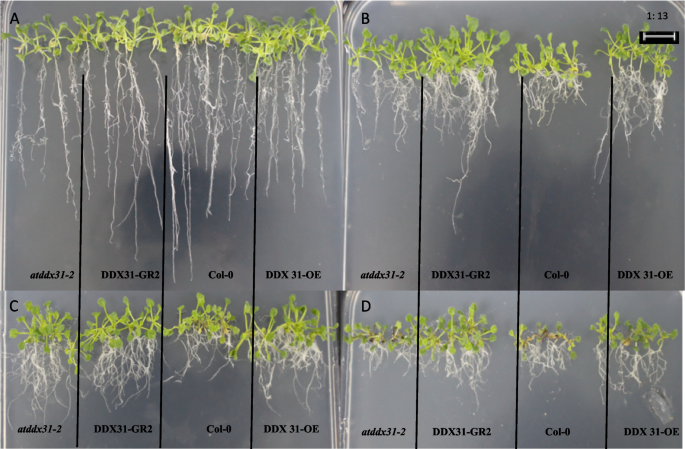
Effects of different mannitol concentrations on atddx31-2 , DDX31-GR2 , Col-0, and DDX31-OE . Note: A-D represent the mannitol medium containing 0 mmol/L, 100 mmol/L, 200 mmol/L, and 300 mmol/L mannitol, respectively
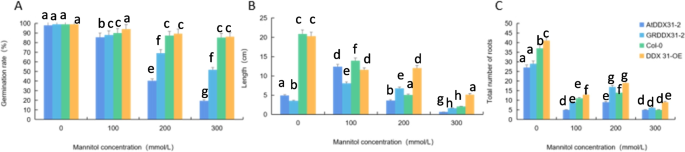
Analysis results of germination rates, root lengths, and numbers of lateral roots of the four types of Arabidopsis thaliana plants corresponding to the RcDDX31 mutant atddx31-2 . Note: Figure A shows the germination rates of A. thaliana plants under drought stress treatments with different mannitol concentrations. Figure B shows the root lengths of A. thaliana plants under drought stress treatments with different mannitol concentrations. Figure C shows the number of lateral roots of A. thaliana plants under drought stress treatments with different mannitol concentrations. Different lowercase letters represent significant differences ( P <0.05)
Analysis of the response of the RcA/HD1 gene to drought stress
To study the response mechanism of the RcA/HD1 gene to drought stress in A. thaliana plants, we phenotypically identified ata/d1 , A/HD1-GR , Col-0, and A/HD1-OE and analyzed the germination rates, root lengths, and numbers of lateral roots under drought stress treatments with different mannitol concentrations. The results are shown in Figs. 39 and 40 . A. thaliana plants grew normally without stress treatment. When A. thaliana plants were treated with 100 mmol/L mannitol, the four types of plants had short root lengths and a limited increase in root number. When A. thaliana plants were treated with 200 mmol/L mannitol, they had shorter root lengths but more lateral roots and chlorotic or reddish-brown leaf abaxial epidermis, and the overexpression and complementary-expression plants had more later roots and longer root lengths. When A. thaliana plants were treated with 300 mmol/L mannitol, they showed weaker overall growth, shorter lateral roots, and reddish-brown leaf abaxial epidermis, but the overexpression and complementary-expression plants had more lateral roots. These findings indicated that the response of this gene to drought stress was mainly manifested in the number of roots and the degree of root elongation.
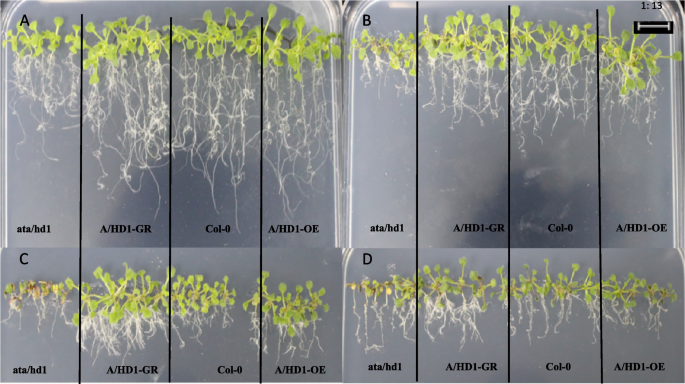
Effect of different mannitol concentrations on ata/hd1, A/HD1-GR , Col-0, and A/HD1-OE . Note: A-D represents the mannitol medium containing 0 mmol/L, 100 mmol/L, 200 mmol/L, and 300 mmol/L, respectively
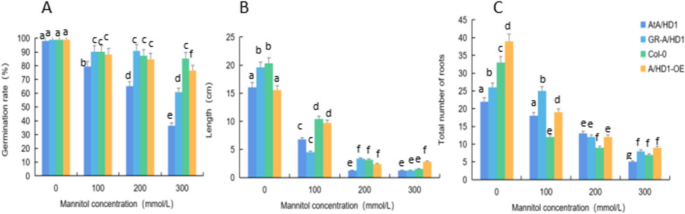
Analysis results of the germination rates, root lengths, and number of lateral roots of the four types of Arabidopsis thaliana corresponding to RcA/HD1 . Note: Figure A shows the germination rates of A. thaliana plants under drought stress treatments with different mannitol concentrations. Figure B shows the root lengths of A. thaliana plants under drought stress treatments with different mannitol concentrations. Figure C shows the numbers of lateral roots of A. thaliana plants under drought stress treatments with different mannitol concentrations. Different lowercase letters represent significant differences ( P <0.05)
Relevant physiological indicators of A. thaliana under drought stress
We measured the physiological indicators (MDA, proline, T-AOC, and hydroxyl radical scavenging ability) of the A. thaliana plants treated with 10% PEG 6000 (drought stress) for 0 h, 24 h, 48 h, and 72 h, with water-treated A. thaliana plants as controls. The measurement results are shown in Figs. 41 , 42 and 43 .
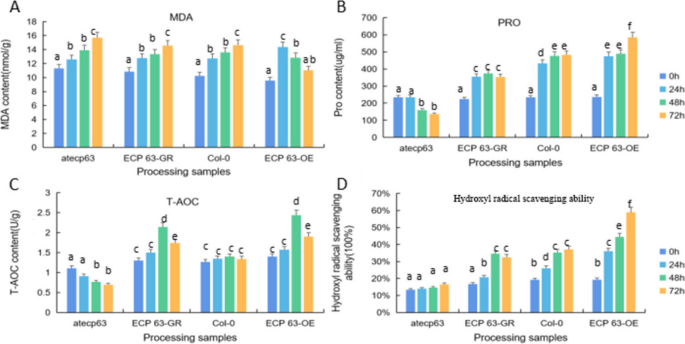
Physiological indicators of four types of Arabidopsis thaliana plants with different RcECP63 genotypes under drought stress treatment with PEG 6000. Note: A: MDA measurement results of atecp63 , ECP63-GR , Col-0, and ECP63-OE at 0 h, 24 h, and 48 h. B: PRO measurement results of atecp63 , ECP63-GR , Col-0, and ECP63-OE at 0 h, 24 h, and 48 h. C: T-AOC measurement results of atecp63 , ECP63-GR , Col-0, and ECP63-OE at 0 h, 24 h, and 48 h. D: Measurement results of hydroxyl radical scavenging ability of atecp63 , ECP63-GR , Col-0, and ECP63-OE at 0 h, 24 h, and 48 h. different lowercase letters indicate significant differences ( P <0.05)
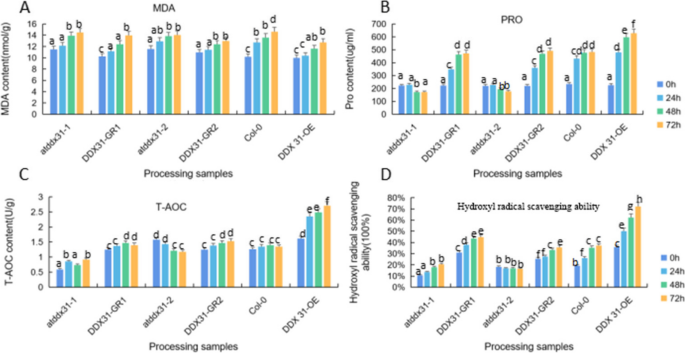
Physiological indicators of four types of Arabidopsis thaliana plants corresponding to the RcDDX31 gene under PEG 6000 stress. Note: A: MDA concentrations of atddx31-1 , DDX31-GR1 , atddx31-2 , DDX31-GR2 , Col-0, and DDX31-OE at 0 h, 24 h, and 48 h. B: Proline concentrations of atddx31-1 DDX31-GR1 , atddx31-2 , DDX31-GR2 , Col-0, and DDX31-OE at 0 h, 24 h, and 48 h. C: T-AOC of atddx31-1 , DDX31-GR1 , atddx31-2 , DDX31-GR2 , Col-0, and DDX31-OE at 0 h, 24 h, and 48 h. D: Hydroxyl radical scavenging ability of atddx31-1 , DDX31-GR1 , atddx31-2 , DDX31-GR2 , Col-0, and DDX31-OE at 0 h, 24 h, and 48 h. Lowercase letters represent significant differences ( P <0.05)
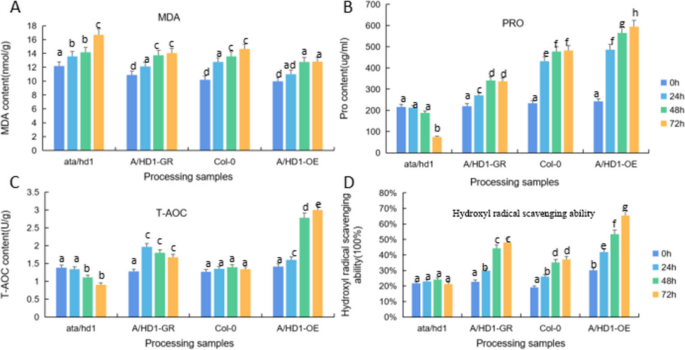
Physiological indicators of four types of Arabidopsis thaliana according to the RcA/HD1 gene under PEG 6000 stress. Note: A: MDA concentration of ata/d1, A/HD1-GR , Col-0, and A/HD1-OE at 0 h, 24 h, and 48 h. B: Proline concentration of ata/d1, A/HD1-GR , Col-0, and A/HD1-OE at 0 h, 24 h, 48 h. C: T-AOC of ata/d1, A/HD1-GR , Col-0, and A/HD1-OE at 0 h, 24 h, and 48 h. D: Hydroxyl radical-scavenging ability of ata/d1, A/HD1-GR , Col-0, and A/HD1-OE at 0 h, 24 h, and 48 h. different lowercase letters represent significant differences ( P <0.05)
Figure 41 shows the measurement results of MDA content, proline content, T-AOC content, and hydroxyl radical scavenging ability of A. thaliana plants with different RcECP63 genotypes at different treatment durations. The results showed that as the stress duration increased, the proline content, hydroxyl radical scavenging ability, and T-AOC content of ECP63-GR , Col-0, and ECP63-OE all significantly increased, while the proline and T-AOC contents of the deletion mutant significantly increased. The MDA and T-AOC contents in the overexpression A. thaliana plants first increased and then decreased. We speculate that the response mechanism of the RcECP63 gene to drought stress is time-dependent and that its activity gradually decreases with increasing treatment duration.
Figure 42 shows the measurement results of MDA content, proline content, T-AOC content, and hydroxyl radical scavenging ability of A. thaliana plants of different RcDDX31 genotypes at different treatment durations. Compared with other types, the overexpression plants had lower MDA contents and the least increase in MDA content. As the stress duration increased, the proline content and hydroxyl radical-scavenging ability increased significantly in the complementary-expression, wild-type, and overexpression plants but remained unchanged or even decreased in the mutant plants, while the T-AOC content showed an overall stable trend in A. thaliana plants but a significant increase in the overexpression plants, which was consistent with the MDA measurement results. The results indicate that overexpressing RcDDX31 enhances the plant drought resistance, which further solidifies the role of RcDDX31 as a drought resistance gene.
Figure 43 shows the measurement results of MDA content, proline content, T-AOC content, and hydroxyl radical scavenging ability of A. thaliana plants of different RcA/HD1 genotypes at different treatment durations. The results showed that as the drought stress increased, the proline content, T-AOC content, and hydroxyl radical scavenging ability of the complementary-expression, wild-type, and overexpression plants increased overall, and these indicators were significantly upregulated in overexpression plants. As the drought stress increased, the MDA content, which reflects the degree of damage, in the complementary-expression, wild-type, and overexpression plants increased but was lower than that in the overexpression plants, and the increment in MDA content in these three types of plants was less than that in the overexpression plants. These results indicate that the transgenic plants responded to external drought stress to adapt to the unfavorable living environment, revealing that RcA/HD1 has drought resistance function.
The above results indicate that overexpressing RcECP63 , RcDDX31 , and RcA/HD1 can make plants more tolerant to drought stress, have a protective effect on plants, reduce the degree of damage to plants, and enhance their antioxidant activity.
The data analysis for each of the following physiological index measurements was carried out using the software SPSS version 19.0 for independent samples t-test, and bar charts were plotted according to the analysis results using WPS software and marked with the significance of differences between samples.
For experimental details see the determination by Yang-Hu Sima [ 9 ].
For experimental details see the determination by A García-Triana [ 10 ].
For experimental details see the determination by Wang L [ 11 ].
For experimental details see the determination by Guo N [ 12 ].
For experimental details see the determination by Naheed Z [ 13 ].
For experimental details see the determination by SE Castrejón [ 14 ].
For experimental details see the determination by Yang Hu Sima [ 9 ].
For experimental details see the determination by Vieira S M [ 15 ].
For experimental details see the determination by Jingang W [ 16 ].
Differential proteomics and widely targeted metabolomic analyses of castor bean embryos at the germination stage
The experimental samples were sent to company for protein extraction, trypsin digestion, iTRAQ labeling, high-performance liquid chromatography (HPLC) fractionation, and liquid chromatography‒mass spectrometry (LC‒MS) analysis, protein characterization, database search, and functional analysis. Proteins with significant differences in expression were selected, and proteins with significant differences in drought-related changes were selected from the differentially upregulated proteins.
The experimental samples were sent to company for sample preparation, screening of differentially expressed metabolites, and data analysis. The enriched metabolic pathways were analyzed to find the differentially expressed metabolites during drought stress. The metabolic pathways responsible for the changes in metabolite levels and their variation patterns were studied.
Combined proteomic and metabolomic analysis
The common metabolic pathways of differentially expressed proteins and differentially expressed metabolites in the same treatment group were identified by differential proteomics and widely targeted metabolomic analysis of the Kyoto Encyclopedia of Genes and Genomes (KEGG)-associated metabolic pathways.
RT-qPCR validation of drought resistance-related genes
The RNA of castor bean embryos was extracted, measured for its OD 260 /OD 280 ratio, and then separated by 1% agarose gel electrophoresis. The RNA solution was reverse-transcribed to obtain cDNA. Eight differentially expressed proteins were selected through differential proteomics, and the mRNAs encoding these proteins were submitted to RT-qPCR analysis to study their expression patterns at the transcriptional level. Expression was normalized to castor bean 18S rRNA gene as an internal reference (Supplementary Table 1 ).
RT-qPCR was performed using the GoTaq® qPCR and RT-qPCR Systems. The qPCR mixes are shown in Supplementary Table 2 . Three biological replicates of each experimental material were used. The average C T was taken to calculate the relative expression levels of the genes using the 2 -ΔΔCт method (Supplementary Table 2 ).
After RT-qPCR of genes encoding differentially expressed proteins, all data were analyzed with SPSS version 19.0 software using independent-sample t test.
The fusion expression vectors PTRV2- RcECP 63 , PTRV2- RcDDX 31 and PTRV2- RcA/HD1 were constructed, and the three target gene fragments were ligated to the PTRV2 vector using the seamless cloning method, respectively, and the constructed silencing vector was transformed into Agrobacterium tumefaciens (OD600=0.6-1.0). The bacterial solution was resuspended and injected into the grate leaves, cultured for 4 d and then treated with 15% PEG 6000 for drought stress, and wild-type castor leaves were used as the control for RNA extraction and RT-qPCR verification.
Functions of differentially expressed genes in A. thaliana
Gene cloning and vector linearization.
The coding sequences of the genes RcECP63 , RcDDX31 , and RcA/HD1 were ligated into the expression vector pCAMBIA-1305.2. The restriction enzyme sites on the gene and on the expression vector pCAMBIA1305.2 were analyzed, and the restriction endonuclease Nco I was finally selected . The primers for seamless cloning of the target genes RcECP63 , RcDDX31 , and RcA/HD1 were designed by using the native software CE Design V1.03 (Supplementary Table 3 ). The three genes were amplified by PCR using Premix PrimeSTAR HS (Supplementary Table 4 ). The reaction conditions were 94 °C for 5 min; 40 cycles of 94 °C for 45 s, T m for 1 min 30 s to 2 min 30 s, 72 °C for 10 min; and hold at 4 °C. After the reaction, the PCR products were detected using 1% agarose gel electrophoresis.
The vector pCAMBIA1305.2 was cleaved using the restriction endonuclease Nco I recognizing a single restriction enzyme site to obtain a linearized vector (Supplementary Table 5 ). The reaction conditions were 37 °C in a water bath for 3 h. After the reaction, the enzyme digestion products were detected by 1% agarose gel electrophoresis.
Ligation of the target genes with the heterologous expression vector
The PCR products and enzyme digestion products of the three target genes that were correctly detected by electrophoresis were recovered for future use. Several target gene fragments and heterologous expression vector fragments were ligated using a seamless cloning kit, and the obtained recombinant vector was used in subsequent experiments (Supplementary Table 6 ). The reaction condition was 50 °C for 1 h.
Transformation of ligation products into competent E. coli cells
Please refer to the study of Yong Zhao [ 17 ] for the specific protocol.
Screening and identification of recombinant E. coli plasmids
The PCR identification system used the universal primers for the vector pCAMBIA1305.2 (Supplementary Table 7 ). the PCR mix is given (Supplementary Table 8 ). After the reaction was completed, the PCR products were subjected to agarose gel electrophoresis, and E. coli with the correct band size was verified by enzyme digestion and sequencing. Plasmids were extracted from E. coli that were confirmed correct by sequencing for transformation of Agrobacterium tumefaciens .
Transformation of Agrobacterium tumefaciens with recombinant plasmid
Screening and identification of recombinant agrobacterium tumefaciens.
The PCR identification system used the universal primers for the vector pCAMBIA1305.2, with the primer sequences and reaction system shown in Supplementary Tables 7 and 8 . After the reaction was completed, the PCR products were subjected to agarose gel electrophoresis, and the Agrobacterium tumefaciens with the correct band size was stored in the preservation solution for subsequent experiments.
Planting, cultivation and transplanting of A. thaliana
Under sterile conditions, an appropriate number of full seeds of Arabidopsis (Col-0, atecp63 , atddx31-1 , atddx31-2 , ata/hd1 ) were transferred to sterile centrifuge tubes. Refer to the study of Yong Zhao for the steps of aseptic planting, culture conditions, later transplanting, and cultivation of A. thaliana [ 9 ].
Genetic transformation of A. thaliana through floral dipping
Agrobacterium tumefaciens containing the RcECP63 , RcDDX31 , and RcA/HD1 genes obtained above was cultured, and the wild-type A. thaliana and the A. thaliana that was mutant in the three genes were genetically transformed through floral dipping.
Screening of transgenic A. thaliana
The seeds of T 0 generation A. thaliana harvested after genetic transformation were dried at room temperature for later use. An appropriate number of seeds were inoculated into Murashige and Skoog selection medium (50 mg/mL hygromycin). A. thaliana was planted in the steps mentioned before. After 25-28 d of culture, A. thaliana seedlings with obvious rooting and good growth were transplanted. When the plants grew to the eight-leaf stage during cultivation, the young leaves were collected for PCR identification. The seeds of the successfully identified plants were harvested for breeding. The homozygous plants were screened according to Mendel's law of heredity, and the T 3 generation A. thaliana plants were selected for phenotypic validation, quantitative analysis, and measurement of physiological indicators.
Identification of drought-resistant overexpression and complementary-expression A. thaliana plants at the molecular level
PCR identification of drought-resistant overexpression and complementary-expression A. thaliana plants: The young leaves (2 mm 2 ) of drought-resistant heterologous-overexpression, and complementary-expression A. thaliana plants were placed in a sterile Eppendorf tube, identified using the M5 HiPer plus Taq HiFi PCR mix, added to 20 μL lysis solution, ground well with a sterile pipette, and boiled for 5 min. After the sample was cooled to room temperature, it was added to 20 μL chloroform. These were mixed by pipetting for 10 s, then centrifuged at 12,000 rpm for 2 min. Two microliters of supernatant was used as the PCR validation template. PCR identification was performed using the universal primers for the vector pCAMBIA1305.2. The primer sequences and reaction system were the same as those in Supplementary Tables 7 and 8 . The reaction conditions were 95 °C for 3 min; 36 cycles of 94 °C for 25 s, T m for 25 s, and 72 °C for 1 min 30 s to 2 min 30 s; and lastly 72 °C for 5 min. After the reaction ended, the PCR products were detected by 1% agarose gel electrophoresis to identify the positive overexpression plants ECP63-OE , DDX31-OE , and A/HD1-OE and the positive complementary-expression plants ECP63-GR . DDX31-GR1 , DDX31-GR2 , and A/HD1GR .
RT-qPCR identification of drought-resistant overexpression and complementary-expression A. thaliana plants: The RNA levels of the overexpression A. thaliana plants ( ECP63-OE , DDX31-OE , and A/HD1-OE ) and the complementary-expression A. thaliana plants ( ECP63-GR , DDX31-GR1 , DDX31-GR2 , and A/HD1-GR ) under drought stress were identified by analyzing the gene expression variation patterns of both sets. The RNA of the overexpression transgenic plants and complementary- expression transgenic plants in the control and treatment groups were extracted and reverse-transcribed to obtain cDNAs. They were submitted to qPCR with AtActin as the internal reference gene. The primer sequences of AtActin, RcECP63 , RcDDX31 , and RcA/HD1 are shown in Supplementary Table 9 .
RT-qPCR identification of resistant overexpression and complementary-expression A. thaliana plants: Different concentrations of PEG and mannitol were used to pretreat the four types (wild-type, mutant, overexpression, and complementary expression) of A. thaliana . To compare the growth status of A. thaliana , the plants were grown in mannitol medium containing 0 mmol/L, 100 mmol/L, 200 mmol/L, and 300 mmol/L mannitol.
Equivalent numbers of homozygous seeds of wild-type, mutant, overexpression and complementary-expression A. thaliana were selected and dibbled in the solid MS medium containing 0 mmol/L, 100 mmol, 200 mmol/L, and 300 mmol/L mannitol after sterilization with 75% alcohol under sterile conditions. After vernalization at 4 °C for 3 d, the samples were cultured in a thermostatic incubator. After germination, the germination rates of different types of seeds in the culture medium containing different mannitol concentrations were recorded. After 14 days of culture, the seedlings were photographed and recorded. The culture conditions were a 16-h/8-h light–dark cycle and a 23/20 °C day/night cycle. After the completion of the culture, we compared the root length and leaf growth of each group of A. thaliana plants and analyzed the root length and the number of lateral roots of the plants expressing RcECP63 , RcDDX31 , and RcA/HD1 under drought stress treatments with different mannitol concentrations.
Measurement of the physiological indicators of four types of A. thaliana under the same treatment conditions
The selected A. thaliana seeds were sterilized and dibbled in the solid MS medium. After vernalization at 4 °C for 3 d, they were transferred to a thermostatic incubator for culture. Fourteen days later, wild-type, deletion-mutant, overexpression, and complementary-expression A. thaliana plants with consistent growth status were transplanted to a medium composed of sterilized nutrient soil:vermiculite at a 3:1 ratio. When the plants reached the eight-leaf stage, they were treated with 10% PEG 6000 (drought stress) for 24 h, 48 h, or 72 h, with water-treated plants as controls, to investigate the changes in four physiological indicators (MDA content, proline content, hydroxyl radical scavenging ability, and T-AOC) of transgenic A. thaliana plants under drought stress.
Discussion and conclusion
The combined proteomic and metabolomic analysis provides more possibilities for exploring and unraveling the complex life activities of organisms. Fan [ 18 ] studied the changes in nutritional quality of alfalfa at different growth stages and found that the common metabolic pathways were mainly enriched in pathways related to nutrient metabolism. Durand Thomas et al. [ 19 ] performed an combined proteomic and metabolomic analysis on A. thaliana seeds and found that the loss of reverse transcriptase stimulates energy metabolism, which in turn affects metabolic pathways, including cell wall biogenesis.
As the end products of gene expression in organisms, metabolites directly or indirectly participate in the metabolic activities of organisms and are most strongly associated with the phenotypes of organisms. Metabolite analyses in Arabidopsis during seed development identified major metabolic abundance transitions associated with successive developmental stages [ 20 ]. The detection of 121 differential metabolites by untargeted metabolomics in Japonica and Indica rice seeds revealed a correlation between metabolic phenotypes and the geographical origin of rice seeds [ 21 ]. Differential metabolite analysis of wheat seeds identified a total of 74 differential metabolites at developmental stages, and further analyses showed that metabolic pathways for biological processes such as amino acids, carbohydrates, and organic acids are interrelated [ 22 ]. Metabolomics provides a reference for better discovering and exploring various complex traits, functions, and features of organisms [ 23 , 24 ]. Seed germination is essentially related to seed metabolism, and the metabolite content and germination ability of seeds are determined by the potential genetic structures and environmental influences during seed development. Sun [ 25 ] found that the vitality and nutritional value of seeds may be related to their chemical compositions. During seed germination, amino acids can play an important role in maintaining osmotic potential.
In this study, the combined proteomic and metabolomic analysis showed that the differentially expressed proteins and differentially expressed metabolites in groups P48_VS_S48 and P60_VS_S60 shared 13 and eight common metabolic pathways, respectively. The combined analysis of the differential proteomics data and comparative metabolomics data of P48_VS_S48 revealed that a total of 13 differential proteins and 13 differential metabolites were annotated to the same metabolic pathways, with the metabolic pathways that shared the same involvement including, Biosynthesis of amino acids, Tyrosine metabolism, 2-Oxocarboxylic acid metabolism, Isoquinoline alkaloid biosynthesis, Carbon metabolism, Phenylalanine metabolism, Phenylalanine, tyrosine and tryptophan biosynthesis, Arginine and proline metabolism, Glyoxylate and dicarboxylate metabolism, Purine metabolism, Tryptophan metabolism, Alanine, aspartate and glutamate metabolism, Citrate cycle, TCA cycle. The combined analysis of the differential proteomics data and comparative metabolomics data of P60_VS_S60 revealed that a total of eight differential proteins and eight differential metabolites were annotated to the same metabolic pathways, and that the metabolic pathways in which the differential proteins and differential metabolites of P60_VS_S60 were jointly involved included Carbon metabolism, Stilbenoid, diarylheptanoid and gingerol biosynthesis, Flavonoid biosynthesis, Citrate cycle, TCA cycle, Biosynthesis of amino acids, Pentose phosphate and gingerol biosynthesis. diarylheptanoid and gingerol biosynthesis, Flavonoid biosynthesis, Citrate cycle, TCA cycle, Biosynthesis of amino acids, Pentose phosphate pathway, Tryptophan metabolism, Glyoxylate and dicarboxylate metabolism.
The physiological significance of the identified common metabolic biological processes, such as the phenylpropanoid biosynthesis, is mainly manifested in their close relationships with physiological activities such as changes in the enzyme system, biosynthesis of intermediate products and their further transformation products, and cell differentiation during plant development. This finding is similar to the findings of previous studies.
In the process of responding to abiotic stress, plants produce drought-induced proteins (LEA, dead box, AK) to adapt to the changing environment. LEA and dead box respond to abiotic stress. Late embryogenesis abundant proteins (LEAs) are a class of proteins that accumulate in large quantities in seeds during the late stages of plant embryogenesis and are an important class of proteins involved in the resistance of organisms to drought stress [ 26 ]. In the 1980s, Dure et al [ 27 ] first reported the presence of LEA genes in developing seeds of cotton. It was found that the high level of LEA protein expression was closely related to the improvement of drought, salt or low temperature tolerance in plants. The isolation of plant LEA genes with higher stress tolerance is of great significance for research in plant adversity stress [ 28 ]. Dead box ATP-dependent RNA helicase is a family of Dead-box ATP-dependent RNA deconjugating enzymes, and the DEAD-box (DDX) family is a family of RNA deconjugating enzymes that are of critical to animal embryo development and has received much attention in recent years. In plants, 32 genes of the DDX deconjugulase family were identified for the first time in Arabidopsis thaliana [ 29 ], followed by several other RNA deconjugulases regulating plant growth and stress [ 30 , 31 , 32 ], which demonstrated that the DDX gene family is involved not only in plant growth and development, but also abiotic stresses such as salt, osmotic, drought stress, cold and phytohormones, including gametophyte and embryo development in Arabidopsis thaliana [ 33 , 34 ]. Aspartate kinase (AK) is a rate-limiting enzyme that restricts the flow rate of carbon and nitrogen sources, and AK is a large class of protein kinases, which has a wide range of applications in fields such as pharmaceutical fermentation, and is one of the key determinants of the ability to obtain high amino acid yields [ 35 ].
Although AK expression is greatly upregulated under stress, there is no relevant research on its role in plant stress resistance. In addition, many protein kinases are highly expressed in response to abiotic stress. In the phenotypic validation of the three differentially expressed genes selected in this study, we found that the common metabolic pathways of the screened differentially expressed proteins and differentially expressed metabolites all play an important role in plant response to biotic stress.
The ideal and commonly used drought stress agents include PEG and mannitol. PEG can be used to simulate soil drought stress [ 36 ]. In this study, mannitol concentrations of 0 mmol/L, 100 mmol/L, 200 mmol/L, and 300 mmol/L were selected to distinguish the phenotypes of different types of A. thaliana plants treated with different concentrations of PEG and different concentrations of mannitol.
In this study, three differentially up-regulated genes, RcECP 63 , RcDDX 31 and RcA/HD1 , were finally selected and analysed for gene function by RT-qPCR analysis of the histological data and selected genes encoding differential proteins. The silencing treatment of castor leaves revealed that castor leaves were more intolerant to drought stress after silencing the three genes, and it was hypothesised that the three selected genes play an important role in the response to drought stress during the germination period of castor. Mannitol tolerance analysis of four types of Arabidopsis thaliana, namely, mutant, back-expressed, wild-type and overexpressed, revealed that the three selected genes were important in the response to drought stress. Laying the foundation for the study of drought tolerance mechanism in castor .
Availability of data and materials
Data and materialshas are all availability.
Li Z, Li HH, Yu SK. Sl WDR204 gene positively regulates drought stress in tomato plants. Anhui Agric Sci. 2019;47(1):96–8.
Google Scholar
Toker C, Canci H, Yildirim T. Evaluation of perennial wild Cicer species for drought resistance. Genet Resour Crop Evol. 2007;54(8):1781–6.
Article Google Scholar
Tuberosa R, Salvi S. Genomics-based approaches to improve drought tolerance of crops. Trends Plant Sci. 2006;11(8):405–12.
Article CAS PubMed Google Scholar
Yao Q. Study on the mechanism of drought stress signalling response of Arabidopsis AtRACK1 protein. Yangzhou University. 2007.
Zhao JD. Effective ways to improve drought resistance of plants. Livestock Feed Sci. 2009; 30(2):50-51+117.
Rodriguez-Cabal HA, Jaramillo-Mazo CY, Franco-Sierra ND, Villanueva-Mejia DF, Alvarez JC. A Differentially Expressed Gene from a High Oil Producer Cultivar of Castor Bean ( Ricinus communis L .) Is Involved in the Biosynthesis of Ricinoleic Acid. Am J Plant Sci. 2020;11(3):393–412.
Article CAS Google Scholar
Cong AQ, Li GR, Wang Y, Li W, Li XC, Huang FL, Chen YS. Cloning and bioinformatics analysis of RcGA20ox1 gene from castor. Mol Plant Breed. 2018;16(10):3121–6.
Huang FL, Peng M, Chen XF, Li GR, Di JJ, Zhao Y, Jiang TT, Chang RH, Yang LF, Chen YS. Comparison of DNA methylation in developing seeds of Ricinus communis L. using MSAP analysis. Biotechnol Biotechnol Equip. 2018;32(4):858–64.
Yang HS, Jin MY, Yi SH, Li W, Lin CZ. Variations of hydrogen peroxide and catalase expression in Bombyx eggs during diapause initiation and termination. Arch Insect Biochem Physiol. 2011;77(2):72–80.
Garcia-Triana A, Zenteno-Savin T, Peregrino-Uriarte AB, Yepiz-Plascencia G. Hypoxia, reoxygenation and cytosolic manganese super- oxide dismutase(cMnSOD) silencing in Litopenaeus vannamei: effects on cMnSOD trans- cripts, superoxide dismutase activity and superoxide anion production capacity. Dev Comp Immunol. 2010;34(11):1230–5.
Wang L, Wang Y, Wang X, Li Y, Peng F, Wang L. Regulation of POD activity by pelargonidin during vegetative growth in radish ( Raphanus sativus L.). Scientia Horticulturae. 2014;174:105–11.
Guo N, Ping X, Xie. A study on the effects of food quantity and quality on glutathione S- transferase (GST) activity and growth rate parameters of Daphnia carinata varying in age. Aquatic Ecol. 2011;45(1):63–73.
Naheed Z, Cheng ZH, Wu CN, Wen YB, Ding HY. Total polyphenols, total flavonoids, allicin and antioxidant capacities in garlic scape cultivars during controlled atmosphere storage. Postharvest Biol Technol. 2017;131:39–45.
SE Castrejón, Yatsimirsky A K. Cyclodextrin enhanced fluorimetric determination of malonal dehyde by the thiobarbituric acid method. Talanta. 1997; 44(6): 951-7.
Vieira SM, Silva TM, Glória MBA. Influence of processing on the levels of amines and proline and on the physico-chemical characteristics of concentrated orange juice. Food Chem. 2010;119(1):7–11.
Wang JG, Wang XK, Guo WL, Guo PQ, Zhong-mao GU. Determination of hydroxyl radicals by methylene blue photometry. Phys Chem Testing. 2007;43(6):3.
CAS Google Scholar
Zhao Y. Drought Resistance of Castor Bean and Functions of Its Receptor-like Protein Kinase. Northeast Forestry University. 2021.
Fan W. Analysis of the variation mechanism of the nutritional quality of alfalfa based on proteomics and metabonomics. Inner Mongolia Agricultural University. 2018.
Durand TC, Cueff G, Godin B, Valot B, Clément G. Combined proteomic and metabolomic profiling of the mutant reveals pleiotropic functions of the retromer in seed development. Int J Mol Sci. 2019;20(2):362.
Article PubMed PubMed Central Google Scholar
Toubiana D, Semel Y, Tohge T, Beleggia R, Cattivelli L, Rosental L, Nikoloski Z, Zamir D, Fernie AR, Fait A. Metabolic profiling of a mapping population exposes new insights in the regulation of seed metabolism and seed, fruit, and plant relations. PLoS Genet. 2012;8:1–23.
Hu Chaoyang, Shi JX, Quan S, Cui B, Kleessen S, Nikoloski Z, Tohge T, Alexander D, Guo L, Lin H, Wang J, Cui X, Rao J, Luo Q, Zhao XX, Fernie AR, Zhang DB. Metabolic variation between japonica and indica rice cultivars as revealed by non-targeted metabolomics. Sci Rep. 2014; 4: 5067-5077.
Zhen SM, Dong K, Deng X, Zhou JX, Xu XX, Han CX, Zhang WY, Xu YH, Wang ZM, Yan YM. Dynamic metabolome profiling reveals significant metabolic changes during grain development of bread wheat ( Triticum aestivum L.). J Sci Food Agric. 2016;96:3731–40.
David T, Wen TX, Nengyi Z, Karl K, Amit G, Shai P, Yves G, Mark S, Edward SB, Alisdair RF, Aaron F. Correlation-based network analysis of metabolite and enzyme profiles reveals a role of citrate biosynthesis in modulating n and c metabolism in zea mays. Front Plant Sci. 2016;7:1–10.
Joosen RVL, Arends D, Yang Li, Willems LAJ, Keurentjes JJB, Ligterink W, Jansen RC, Hilhorst HWM. Identifying genotype-by-environment interactions in the metabolism of germinating arabidopsis seeds using generalized genetical genomics. Plant Physiol. 2013; 162(2): 553-566.
Sun Y. Functions of the genes in the same families of transcription factors OsbZIP18 and OsBCAT in rice. Huazhong Agricultural University. 2020.
Hua YR. Cloning and functional expression study of ALDH gene family of acetaldehyde dehydrogenase from Olea europaea. Central South Forestry University of Science and Technology. 2017.
Dure L, Greenway SC, Galau GA. Developmental biochemistry of cottonseed embryogenesis and germi nation: changing messenger ribonucleic acid populations as shown by in vitro and in vivo protein synthesis. Biochemistry. 1981;20(14):4162–8.
Hand SC, Menze MA, Toner M, Boswell L, Moore D. LEA proteins during water stress: not just for plants anymore. Ann Rev Physiol. 2011;73(1):115–34.
Aubourg S, Kreis M, Lecharny A. The DEAD-box RNA helicase family in Arabidopsis thaliana. Nucleic Acids Res. 1999;27(2):628–36.
Article CAS PubMed PubMed Central Google Scholar
Gong ZZ, Dong CH, Lee HJ, Zhu JH, Xiong LM, Gong DM, Stevenson B, Zhu JK. A DEAD-box RNA helicase is essential for mRNA export and impor- tant for development and stress responses in Arabidopsis. Plant Cell. 2005;17:256–67.
Tripurani SK, Nakaminami K, Thompson KB, Crowell SV, Guy CL, Karlson DK. Spatial and temporal expression of cold-responsive DEAD- box RNA helicases reveals their functional roles during embryogenesis in Arabidopsis thaliana. Plant Mol Biol Rep. 2011;29(4):761–8.
Takagi M, Iwamoto N. Arabidopsis SMN2/HEN2, Encoding DEAD-box RNA Helicase, Governs proper expression of the resistance gene SMN1/RPS6 and is involved in dwarf, autoimmune phenotypes of mekk1 and mpk4 Mutants. Plant Cell Physiol. 2020.
Khan A, Garbelli A, Grossi S, Florentin A, Batelli G, Acuna T, Zolla G, Kaye Y, Paul LK, Zhu JK. The Arabidopsis STRESS RESPONSE SUPPRESSOR DEAD-box RNA helicases are nucleolar and chromocenter-localized proteins that undergo stress-mediated relocalization and are involved in epigenetic gene silencing. Plant J Cell & Mol Biol. 2014;79(1):28–43.
Nguyen LV, Seok HY, Woo DH, Lee SY, Moon YH. Overexpression of the DEAD-Box RNA helicase gene AtRH17 confers tolerance to salt stress in Arabidopsis. Int J Mol Sci. 2018;19(12):3777.
Yang Y-T. Effects of fixed-point mutations in aspartate kinase S381 and E382 of Corynebacterium pekinense on enzyme activity and characterisation of enzymatic properties. Jilin Agricultural University. 2016.
Carpita N, Sabularse D, Montezinos D, Delmer D, P. Determination of the Pore Size of Cell Walls of Living Plant Cells. Science. 1979; 205(4411): 1144-1147.
Download references
National Natural Science Foundation of China (31860071); Ministry of Education New Agricultural Research and Reform Practice Project (2020114); Upper Level Project of Inner Mongolia Natural Science Foundation (2021MS03008); Inner Mongolia Autonomous Region Grassland Talent Innovation Team - Rolling Support Programme for Castor Molecular Breeding Research Innovation Talent Team (2022); 2021 Research Project on Higher Education Teaching Reform of the National People's Committee (21082); Inner Mongolia Autonomous Region Science and Technology Department set up the project of construction of key laboratory of castor breeding and comprehensive utilization in Inner Mongolia Autonomous Region; Inner Mongolia University for Nationalities 2022 Basic Research Operating Expenses of Colleges and Universities directly under the Autonomous Region (237); Inner Mongolia University for Nationalities 2023 Basic Research Operating Expenses of Colleges and Universities directly under the Autonomous Region (225, 227, 243, 244,); Project of the Open Fund of the Collaborative Innovation Centre for Castor Industry in Inner Mongolia Autonomous Region (MDK2021011, MDK2022014); Analysis of the R2R3-MYB transcription factor gene family in castor bean and functional resolution of RcMYB61 in response to drought stress (2021BS03036).
Author information
Zhiyan Wang and Rui Luo made equal contributions to this study. They are co-first authors.
Authors and Affiliations
College of Life Science and Food Engineering, Inner Mongolia Minzu University, Tongliao, 028000, Inner Mongolia, China
Zhiyan Wang, Rui Luo, Qi Wen, Mingda Yin, Yanpeng Wen & Xuemei Hu
Agronomy College, Jilin Agricultural University, Changchun, 130118, China
Xiaotian Liang
National Oat Improvement Center, Baicheng Academy of Agricultural Sciences, Baicheng, 137000, China
China National Rice Research Institute, Hangzhou, 311400, China
School of Life Sciences, Laboratory of Genetics, Baicheng Normal University, Baicheng, 137000, China
Key Laboratory of Castor Breeding of the National Ethnic Affairs Commission of the People’s Republic of China, Tongliao, 028000, Inner Mongolia, China
Fenglan Huang
Inner Mongolia Key Laboratory of Castor Breeding and Comprehensive Utilization, Tongliao, 028000, Inner Mongolia, China
You can also search for this author in PubMed Google Scholar
Contributions
(This statement must exactly match on Editorial submission system and in the manuscript):Zhiyan Wang and Rui Luo are the authors of this article; Qi Wen, Xiaotian Liang, Huibo Zhao, Yong Zhao, Yanpeng Wen, Mingda Yin, and Xuemei Hu were the summary organisers of the data and literature; Fenglan Huang was the supervisor of the paper writing and revision. All authors read and agreed to the final text. For experiments involving human participants (including the use of tissue samples), informed consent must have been obtained: inapplicable
Corresponding author
Correspondence to Fenglan Huang .
Ethics declarations
Ethics approval and consent to participate.
Approval ethics and consent to participate.
Consent for publication
Not applicable.
Competing interests
The authors declare no competing interests.
Additional information
Publisher's note.
Springer Nature remains neutral with regard to jurisdictional claims in published maps and institutional affiliations.
Supplementary Information
Supplementary material 1., supplementary material 2., rights and permissions.
Open Access This article is licensed under a Creative Commons Attribution 4.0 International License, which permits use, sharing, adaptation, distribution and reproduction in any medium or format, as long as you give appropriate credit to the original author(s) and the source, provide a link to the Creative Commons licence, and indicate if changes were made. The images or other third party material in this article are included in the article's Creative Commons licence, unless indicated otherwise in a credit line to the material. If material is not included in the article's Creative Commons licence and your intended use is not permitted by statutory regulation or exceeds the permitted use, you will need to obtain permission directly from the copyright holder. To view a copy of this licence, visit http://creativecommons.org/licenses/by/4.0/ . The Creative Commons Public Domain Dedication waiver ( http://creativecommons.org/publicdomain/zero/1.0/ ) applies to the data made available in this article, unless otherwise stated in a credit line to the data.
Reprints and permissions
About this article
Cite this article.
Wang, Z., Luo, R., Wen, Q. et al. Screening and functional verification of drought resistance-related genes in castor bean seeds. BMC Plant Biol 24 , 493 (2024). https://doi.org/10.1186/s12870-024-04997-7
Download citation
Received : 01 September 2023
Accepted : 08 April 2024
Published : 03 June 2024
DOI : https://doi.org/10.1186/s12870-024-04997-7
Share this article
Anyone you share the following link with will be able to read this content:
Sorry, a shareable link is not currently available for this article.
Provided by the Springer Nature SharedIt content-sharing initiative
- Castor bean
- Drought stress
BMC Plant Biology
ISSN: 1471-2229
- General enquiries: [email protected]
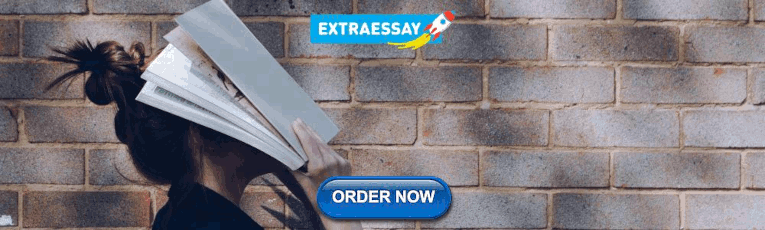
COMMENTS
Plant Biology is an international journal of broad scope bringing together different subdisciplines, such as physiology, molecular biology, cell biology, development, ... Duckweed - Research and Application . January 2014 Special Issue: Plant Biology in Space . January 2013 Special Issue:
Research - Plant Biology Humankind depends on plant growth and productivity not only for human sustenance, but also for alternatives to fossil fuel or nuclear energy. In addition, plants are important for climate stability, and are a key resource for discovering new macromolecules that have applications in medicine and other important fields.
News & Views 22 May 2024 Nature Genetics. P: 1-3. Plant ecology. Plant evolution. Plant genetics. Plant hormones. Plant immunity. Plant molecular biology. Plant physiology.
Journal of Plant Biology is an international journal dedicated to essential research in various fields of plant science. Recognized for detailing significant research contributions to plant science. Broad coverage, including biotechnology, biochemistry, and macromolecular structure. Focuses on cellular and developmental biology, ecology ...
Aims and scope. BMC Plant Biology is an open access, peer-reviewed journal that considers articles on all aspects of plant biology, including molecular, cellular, tissue, organ and whole organism research.
Nature Plants is a scientific journal publishing primary research papers concerned with all aspects of plant biology, technology, ecology and evolution.
Plant biology research is positioned to play a central role in this critical endeavor. It is an exciting and urgent time to be—or become—a plant scientist. Author Contributions. The author confirms being the sole contributor of this work and has approved it for publication.
Overview. Journal of Plant Research is an international publication focusing on fundamental knowledge in all areas of plant sciences. Encourages work based on unique approaches and unprecedented findings. Welcomes interdisciplinary studies and newly developing areas of basic plant biology. Papers should be hypothesis-driven, not purely descriptive.
Plant cell biology is the study of all aspects of plant cells. It is particularly concerned with structure, growth, division, signalling, differentiation and death of plant cells. The nuclear pore ...
About the journal. This journal aims to acknowledge and encourage interdisciplinary research in fundamental plant sciences with scope to address crop improvement, biodiversity, nutrition and human health. It publishes review articles, original research papers, method papers and short articles in plant research …. View full aims & scope.
ISSN: 1543-5008. eISSN: 1545-2123. AIMS AND SCOPE OF JOURNAL: The Annual Review of Plant Biology covers the significant developments in the field of plant biology, including biochemistry and biosynthesis, genetics, genomics and molecular biology, cell differentiation, tissue, organ and whole plant events, acclimation and adaptation, and methods ...
The Eceriferum ( CER) gene family is associated with wax production and stress resistance. Rasmieh Hamid, Zahra Ghorbanzadeh, Feba Jacob, Mojtaba Khayam Nekouei, Mehrshad Zeinalabedini, Mohsen Mardi, Akram Sadeghi and Mohammad Reza Ghaffari. BMC Plant Biology 2024 24 :468. Research Published on: 29 May 2024.
The Plant Biology Initiative at Harvard University. Harvard University is home to a wide range of plant biologists, primarily situated in the Department of Organismic and Evolutionary Biology and the research programs of the Arnold Arboretum and the Harvard Forest.These researchers apply a diverse array of tools and approaches to investigate questions in plant evolution, ecology, physiology ...
Gilbert Building 371 Jane Stanford Way Stanford, CA 94305 Phone: 650-723-2413 biologyinfo [at] stanford.edu (biologyinfo[at]stanford[dot]edu) Campus Map
Plant genes serve as an instruction book for plant function—controlling everything from reproduction to growth to metabolism. At Salk, researchers catalog those genetic instructions to better understand plant function and dysfunction, as well as how those instructions could be altered to create more resilient plants in the future. News Faculty.
The Department of Plant and Microbial Biology at UC Berkeley consistently ranks among top institutions worldwide for research and training in plant biology and microbiology. Learn more » Research. In the fields of biochemistry, plant genetics, genomics, host-microbe interactions, biotechnology, ecology, and much more, our department is making ...
The American Society of Plant Biologists, founded in 1924 to promote the growth and development of plant biology, to encourage and publish research.
The fertility of modern plant-biology research is demonstrated in special issues of Science (November 16, 1990) and Cell (January 27, 1989). Developmental biology, cell-to-cell recognition, signal transduction, the molecular basis of disease, plant-microbe interactions, gene regulation, transposition, and photosynthesis are some of the areas ...
The Plant Biology Section is home to some of the most influential plant science research in the world. The Section represents diverse intellectual interests and boasts a collaborative community culture. Including emeritus professors, there are three members of the National Academy of Sciences. Undergraduates, graduate students, and post-doctoral researchers can join field-leading faculty as ...
The most cited plant science journal advances our understanding of plant biology for sustainable food security, functional ecosystems and human health. ... Submit your research. Start your submission and get more impact for your research by publishing with us. Author guidelines.
Plant biotechnology articles from across Nature Portfolio. ... Research Open Access 30 May 2024 Nature Communications. Volume: 15, P: 4610 ... Research Open Access 20 May 2024 Communications Biology.
International Journal of Plant Biology is an international, peer-reviewed, open access journal on all different subdisciplines of plant biology, published quarterly online by MDPI (from Volume 13, Issue 1 - 2022).. Open Access — free for readers, with article processing charges (APC) paid by authors or their institutions.; High Visibility: indexed within Scopus, Biological Abstracts and ...
The Plant Cell publishes novel research of special significance in plant biology, especially in the areas of cellular biology, molecular biology, biochemistry, genetics, development, and evolution.
Crop breeders will be able to overcome the difficulties they face by utilizing various breeding methods based on plant physiology, genetics and molecular research accumulated so far. Recently, in crop breeding, the development of omics and gene editing technology has made it possible to precisely manipulate target genes, enabling the breeding ...
Space Biology research helps us understand the fundamentals of plant growth by examining the very building blocks of plant life down to the molecular level: transcriptomics, genomics, proteomics, and metabolomics. To compare the effects of microgravity conditions on plants, we also conduct experiments on Earth using gravity or simulated ...
RESEARCH. Plant biology research is incredibly diverse starting at soil and microbial interactions, to whole plant systems and genomes, to how the molecules and genes interact, to gene manipulation, to ecosystems and evolution. Our faculty's research issues address climate change, RNA mediated processes to understand genetic and biochemical ...
The Salk Institute For Biological Studies: Unlocking the secrets of life itself is the driving force behind the Salk Institute. Our team of world-class, award-winning scientists pushes the boundaries of knowledge in areas such as neuroscience, cancer research, aging, immunobiology, plant biology, computational biology and more.
Postdoctoral Research Fellowship in Plant Biology. The proposed research will contribute to fundamental understanding of the role plant growth regulators or hormones play in mediating plant growth and differentiation. The objective is to clone and characterize the gene (s) for 1- aminocyclopropane-1-carboxylic acid (ACC) synthase in tomato.
Drought is one of the natural stresses that greatly impact plants. Castor bean (Ricinus communis L.) is an oil crop with high economic value. Drought is one of the factors limiting castor bean growth. The drought resistance mechanisms of castor bean have become a research focus. In this study, we used castor germinating embryos as experimental materials, and screened genes related to drought ...