An IoT-Based Water Management System for Smart Cities
- Conference paper
- First Online: 23 February 2021
- Cite this conference paper
- Immanuel Savio Donbosco 11 &
- Udit Kr. Chakraborty 11
Part of the book series: Lecture Notes in Civil Engineering ((LNCE,volume 115))
338 Accesses
3 Citations
Water conservation is one of the prime concerns in the current scenario where environmental conditions are deteriorating at an alarming rate. Smart cities, unlike the conventional system of living, are in the frontline of innovation in terms of both connectivity and technological advancement. The main idea is to use the available technology to make life easy with lesser harm to the environment. An Internet of Things (IoT) and data analytics (DA) based water management system will be a basic ground for implementation and future research on how data and IoT can be used to make this happen. This paper proposes an Internet of Things (IoT) and data analytics (DA) based water distribution cum management system that would help in optimal distribution of water based on user consumption at the plot holding level. The proposed system would not only save water misuse but also help in storing usage data for analysis and town planning at a macro-level.
This is a preview of subscription content, log in via an institution to check access.
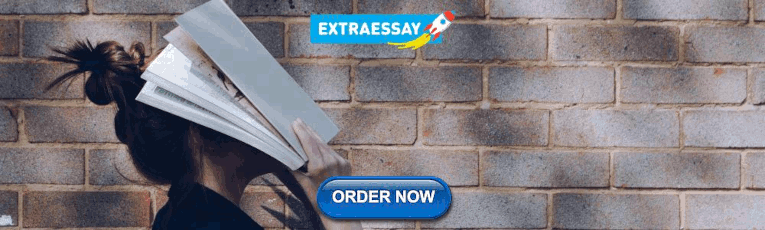
Access this chapter
- Available as PDF
- Read on any device
- Instant download
- Own it forever
- Available as EPUB and PDF
- Compact, lightweight edition
- Dispatched in 3 to 5 business days
- Free shipping worldwide - see info
- Durable hardcover edition
Tax calculation will be finalised at checkout
Purchases are for personal use only
Institutional subscriptions
Similar content being viewed by others
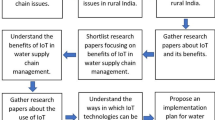
Framework for the implementation of an Internet of Things (IoT)-based water distribution and management system
IoT as an Assistive Technology for Community-Based Water Management Practices During COVID-19 Pandemic and Beyond
Automated Water Management System Using Internet of Things
Mohanasundaram SV, Joyce A, Naresh SK, Gokulakrishnan G, Kale A, Dwarakanath T, Haribabu P (2018) Smart water distribution network solution for smart cities: Indian scenario. 2018 Global Internet of Things Summit (GIoT). https://doi.org/10.1109/GIOTS.2018.8534524
Agarwal N, Agarwal G Role of cloud computing in development of smart city. Int J Sci Technol Eng (IJSTE), (online) ISSN: 2349-784X
Google Scholar
Merchanta A, Mohan Kumar MS, Ravindra PN, Vyas P, Manohar U (2013) Analytics driven water management system for Bangalore city. In: 12th International conference on computing and control for the water industry, CCWI2013, Procedia Engineering, vol 70, pp 1137–1146
Karwot J, Kaźmierczak J, Wyczolkowski R, Paszkowski W, Przystałka P (2016) Smart water in smart city: a case study, conference: SGEM 16th international scientific conference on earth and Geosciencesat: Albena, Bulgaria vol 3/I. https://doi.org/10.5593/sgem2016B31 .
Kaur MJ, Maheshwari P (2016) Building smart cities applications using IoT and cloud-based architectures. In: Proceedings of 2016 international conference on industrial informatics and computer systems (CIICS). https://doi.org/10.1109/GIOTS.2018.8534524 .
Su K, Li J, Fu H (2011) Smart city and the applications. In: Proceedings of the 2011 international conference on electronics, communications and control (ICECC). https://doi.org/10.1109/ICECC.2011.6066743
Koo D, Piratla K, John Matthews C (2015) Towards sustainable water supply: Schematic development of big data collection using internet of things (IoT). Procedia Eng 118:489–497
Karwati K, Kustija J (2018) Prototype of water level control system. In: International symposium on materials and electrical engineering (ISMEE) 2017, materials science and engineering 384:012032. https://doi.org/10.1088/1757-899X/384/1/012032
Priya R, Rameshkumar GP (2017) A novel method to smart city’s water management system with sensor devices and arduino. Int J Comput Intell Res 13(10):2391–2406 ISSN 0973-1873
Varun KS, Kumar KA, Chowdary VR, Raju CSK (2018) Water level management using ultrasonic sensor (automation). Int J Comput Sci Eng 6(6):799–804. E-ISSN: 2347-2693
Paska D (2018) Digitalized water and smart cities—how can telecommunication networks be used for environmental resilience? International Telecommunication Union, 2018, ICT Discoveries, Special Issue No. 2
https://www.hackster.io/jeffpar0721/add-wifi-to-arduino-uno-663b9e
Mulyana Y, Hakim DL (2017) Prototype of water turbidity monitoring system. In: Proceedings of the international symposium on materials and electrical engineering (ISMEE). https://doi.org/10.1088/1757-899X/384/1/0120512
Karray F, Triki M, Jmal MW, Abid M, Obeid AM (2018) WiRoTip: an IoT-based wireless sensor network for water pipeline monitoring. Int J Electr Comput Eng (IJECE) 8(5):3250–3258, ISSN: 2088-8708. https://doi.org/10.11591/ijece.v8i5.pp3250-3258
Immanuel SD, Chakraborty (2019) UK Genetic algorithm: An approach on optimization. In: 2019 International Conference on Communication and Electronics Systems (ICCES), Coimbatore, India, pp 701–708. https://doi.org/10.1109/ICCES45898.2019.9002372
Download references
Author information
Authors and affiliations.
Department of Computer Science and Engineering, Sikkim Manipal Institute of Technology, Majitar, Sikkim, India
Immanuel Savio Donbosco & Udit Kr. Chakraborty
You can also search for this author in PubMed Google Scholar
Corresponding author
Correspondence to Immanuel Savio Donbosco .
Editor information
Editors and affiliations.
Sikkim Manipal Institute of Technology, Sikkim, India
Chandrashekhar Bhuiyan
Friedrich Schiller University, Jena, Jena, Thüringen, Germany
Wolfgang-Albert Flügel
Department of Civil Engineering, Indian Institute of Technology Roorkee, Roorkee, India
Sharad Kumar Jain
Rights and permissions
Reprints and permissions
Copyright information
© 2021 Springer Nature Singapore Pte Ltd.
About this paper
Cite this paper.
Donbosco, I., Chakraborty, U.K. (2021). An IoT-Based Water Management System for Smart Cities. In: Bhuiyan, C., Flügel, WA., Jain, S.K. (eds) Water Security and Sustainability. Lecture Notes in Civil Engineering, vol 115. Springer, Singapore. https://doi.org/10.1007/978-981-15-9805-0_21
Download citation
DOI : https://doi.org/10.1007/978-981-15-9805-0_21
Published : 23 February 2021
Publisher Name : Springer, Singapore
Print ISBN : 978-981-15-9804-3
Online ISBN : 978-981-15-9805-0
eBook Packages : Engineering Engineering (R0)
Share this paper
Anyone you share the following link with will be able to read this content:
Sorry, a shareable link is not currently available for this article.
Provided by the Springer Nature SharedIt content-sharing initiative
- Publish with us
Policies and ethics
- Find a journal
- Track your research

An official website of the United States government
The .gov means it’s official. Federal government websites often end in .gov or .mil. Before sharing sensitive information, make sure you’re on a federal government site.
The site is secure. The https:// ensures that you are connecting to the official website and that any information you provide is encrypted and transmitted securely.
- Publications
- Account settings
Preview improvements coming to the PMC website in October 2024. Learn More or Try it out now .
- Advanced Search
- Journal List
- Sensors (Basel)

Smart Technologies for Water Resource Management: An Overview
Stefania anna palermo.
1 Department of Civil Engineering, University of Calabria, 87036 Rende, CS, Italy
Mario Maiolo
2 Department of Environmental Engineering, University of Calabria, 87036 Rende, CS, Italy
Anna Chiara Brusco
Michele turco, behrouz pirouz, emilio greco.
3 CNR-National Research Council of Italy, Institute for High Performance Computing and Networking (ICAR), 87036 Rende, CS, Italy
Giandomenico Spezzano
Patrizia piro.
The latest progress in information and communication technology (ICT) and the Internet of Things (IoT) have opened up new opportunities for real-time monitoring and controlling of cities’ structures, infrastructures, and services. In this context, smart water management technology provides the data and tools to help users more effectively manage water usage. Data collected with smart water devices are being integrated with building management systems to show how much water is used by occupants as well as to identify the consumption areas to use water more efficiently. By this approach, smart buildings represent an innovative solution that enhances a city’s sustainability and contributes to overcoming environmental challenges due to increasing population and climate change. One of the main challenges is resource-saving and recovery. Water is an all-important need of all living beings, and the concerns of its scarcity impose a transition to innovative and sustainable management starting from the building scale. Thus, this manuscript aims to provide an updated and valuable overview for researchers, consumers, and stakeholders regarding implementing smart and sustainable technologies for water resource management, primarily for building-scale uses.
1. Introduction
The increase in population raised human demand, and overuse of water for domestic, agricultural, commercial, and industrial purposes—combined with climate change and pollution—is a serious issue affecting the sustainability of the environment. Since water is a limited natural resource, its proper use and management are crucial [ 1 ].
In this context, monitoring water usage in different sectors for better management is one of the aspects that is taken into account in smart city development, which is one of the subjects that has garnered significant interest in the last few years. The development of this innovative concept to improve cities is principally due to the recent progress in information and communication technologies (ICT) and especially the Internet of things (IoT).
Moving towards more intelligent management of the urban environment enhances the quality of human life and increases sustainability. The development of new systems contradicts the background of cyber-physical systems, big data, and digitalization, where processors, sensors, software, communication, and control devices are all integrated to enable informed decisions in an increasingly changing, uncertain, and complex environment. Although a unique definition of this concept is still lacking, a smart city includes many aspects (institutional, technical, social, and economic) in interaction with urban infrastructures [ 2 , 3 ]. Thus, as reported in [ 4 ], there are six core areas or aspects that form the concept of smart cities: smart economy (competitiveness); smart governance (citizen participation); smart people (social and human capital); smart mobility (transport and ICT); environment (natural resources); and smart living (quality of life).
All of these features have to be taken into consideration starting from the development of buildings that need to be smart, i.e., able to properly manage resources and provide the best possible comfort to inhabitants. In this regard, the term “intelligent building” was born in the 1980s in the United States to indicate a building with sophisticated devices for management and data network services. More in detail, the definition of building intelligence focused on building automation systems and solutions, known as building management systems (BMS) [ 5 ]. Over the past three decades, advances have been remarkable, thanks to technology’s significant evolution, the advent of the Internet, IoT, and hardware and software solutions more generally.
Overall, in a smart building, the optimization of resources takes a key role in reducing their consumption and increasing the sustainability of the building. Thus, sensor devices within smart buildings enable smart water consumption management, early and fast detection of cracks in pipes, and monitoring of water consumption and leakage in individual building segments. Water meters transmit data to a centralized system that stores water consumption information, draws conclusions about possible water leaks, sends alarms to the user or a system, and implements machine learning algorithms with the goal of possible savings and detection of minor and/or consumption trends.
Generally, information on water level, flow, and pressure is crucial in order to identify the best operational management strategy, providing an efficient and cost-effective solution through broader water resource monitoring [ 6 ].
Thus far, many reviews and surveys in the literature have analyzed smart water monitoring and management systems. In 2013, Boyle et al. [ 7 ] reviewed the devices and global development of intelligent metering for urban water. In another review article [ 8 ], the authors presented a survey focused on IoT-based smart water quality monitoring systems specially dedicated to domestic applications. Similarly, Geetha and Gouthami [ 9 ] have presented a detailed overview of smart water quality monitoring. While in [ 10 ], the authors discussed the architecture and the components of IoT-based systems (water level and water quality monitoring systems). Saad et al. [ 11 ] presented a survey on water management and monitoring in agriculture. Recently, Ismail et al. [ 12 ] focused on smart IoT-based water management and monitoring system for several applications (agricultural, industrial, and residential). In another study [ 13 ], an overview of the water monitoring systems—from traditional techniques to IoT-based monitoring—was carried out by focusing primarily on the IoT-controlled water storage tanks (IoT-WST). Oberascher et al. [ 2 ] provided a guideline for communication technologies for monitoring and controlling urban water infrastructures. Vijayan et al. [ 14 ] have analyzed basic services in smart buildings (security control, energy management, control and monitoring of HVAC systems, water management, lighting systems, fire detection, health systems, and elderly care) to provide a guideline for future research. Other reviews focused on specific methods for leakage detection and localization in pipelines. In this regard, Adedeji et al. [ 15 ] have provided a comprehensive survey of developments in leakage detection and localization methods, while Colombo et al. [ 16 ] focused on transient-based leak methods and Mohd Ismail et al. [ 17 ] explored vibration detection methods using accelerometer sensors.
Starting from the previous review of studies and surveys and given the key role of these advanced tools in saving water resources, this paper presents an overview of innovative systems to support the widespread use of smart and sustainable technologies for water management. Therefore, the main objective of this manuscript is to provide an update and valuable overview for researchers, consumers, and stakeholders regarding the implementation of smart and sustainable technologies in the field of water resource management. The novelty compared with the other reviews is that this study focuses on smart water monitoring and management primarily at the building scale by investigating IoT-based systems and strategies for measuring water levels, monitoring water consumption, and detecting leaks.
The paper is structured as follows. Section 2 presents the research background on the advent of the Internet of Things (IoT) technologies and an introduction to the smart building concept. Section 3 reviews the sensors used for smart water management—primarily in buildings; focusing on water level, water consumption, and leakage detection sensors. While Section 4 illustrates some sustainable systems for water-saving and management that can be integrated into the smart building concept. Section 5 presents an in-depth discussion about the analyzed systems, current challenges, and future directions of IoT-based smart water monitoring and control systems. Section 6 is left for the work’s main conclusions.
2. Research Background
2.1. internet of things (iot).
The definition of the “Internet of Things” was first used in 1999 by Kevin Aston, an engineer and researcher at the Massachusetts Institute of Technology in Cambridge. He referred to the IoT as uniquely identifiable interoperable connected objects with radio-frequency identification (RFID) technology [ 18 ]. The words “Internet” and “Things” denote an interconnected global network based on sensory, communication, networking, and information processing technologies, which could be the new version of information and communication technology (ICT) [ 19 , 20 ].
IoT devices embedded in these physical objects fall mainly into switches (which send a command to a thing) or sensors (which acquire data and send them elsewhere). This way, information regarding physical elements can be shared, and data collection can be completed through low-cost computing, big data, cloud, analytics, and mobile technologies, with minimal human intervention. Thus, the physical world meets and cooperates with the digital world, creating a hyperconnected world with digital systems that can record, monitor, and regulate every interaction between connected objects [ 21 , 22 , 23 ].
A typical IoT system functions by creating a continuous feedback loop. Depending on the IoT system type, analysis can be performed by physical intervention or artificial intelligence and machine learning (AI/ML) technologies, either near real-time or long-term. Despite the IoT definition changes depending on different deployment technologies, the foundation of IoT implies that objects in an IoT can be uniquely identified in virtual representations. Within an IoT, all objects can exchange data and, if necessary, process them according to predefined patterns [ 21 , 24 ].
The stages of IoT evolution started with RFID technology, which is increasingly used in logistics, pharmaceutical manufacturing, logistics, and different industries [ 25 , 26 , 27 ]. Thus, emerging wireless sensor technologies have extended the original IoT concept to ambient intelligence and autonomous control (wireless sensor networks (WSNs), barcodes, smart sensing, RFID, NFC, low-energy wireless communications, and cloud computing) [ 28 , 29 , 30 , 31 ]. The evolution of these technologies brings new technologies to the IoT [ 32 , 33 , 34 ].
The main architecture of IoT includes three layers: the physical layer, the network layer, and the application layer. At the physical layer, sensors collect and transform data from the external environment. Time-sensitive data can be processed simultaneously and collected or stored in the cloud [ 35 ]; specifically, data are collected at the network layer and transformed for data processing [ 36 ]. Finally, the application layer is responsible for providing specific services to the user.
As reported in [ 37 ], the integration of the IoT paradigm into water management processes can provide several benefits—such as improving water management infrastructures by increasing their efficiency, reducing energy cost and human intervention; reducing water management costs; enhancing asset utilization by using sensors and connectivity; and increasing productivity—thereby expanding new and existing business models, based on the three pillars of the IoT (internet-oriented; thing-oriented; knowledge-oriented).
Following the advances in IoT technologies, smart infrastructures are increasingly becoming self-monitored, self-communicated, and especially self-administered. Several factors have enabled this transition, including sustainability, resources management, economics, rapid development of information technology, and advances in computing and communication systems [ 23 ].
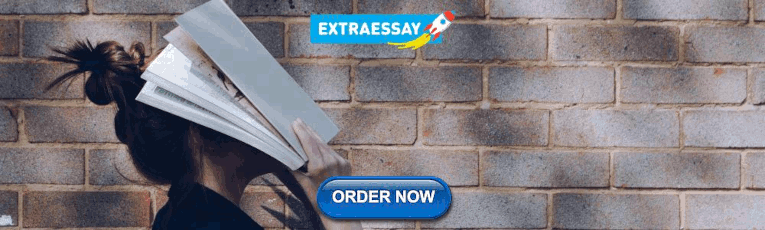
2.2. Smart Building
From its earliest forms, ‘intelligent buildings’—also known as ‘smart buildings’—have had many definitions. As early as 1981, the United Technology Building Systems Corporation of the USA (UTBS Corporation) first used the term “intelligent building”, and two years later, the City Place Building in Hartford, Connecticut, became known worldwide as the first intelligent building [ 38 ]. The European Commission has provided a “smart building” specific definition, according to which a smart building includes communication technologies able to link different objects, sensors, and functions, allowing them to communicate with each other and be controlled remotely [ 39 ]. Moreover, a smart building can take full advantage of efficiency, realizing resources capable of managing and containing general costs [ 40 ]. Based on these definitions, smart building central systems work thanks to digital platforms and electronic sensors. In addition to the timely detection of parameters, these systems can communicate in an automated and integrated way through supervision and control software infrastructure. This type of management makes buildings safer, more efficient, and greener.
Thus, for both private and public use, a smart building is an ecosystem capable of offering different services, divided into six areas: energy, safety, security, comfort, health, and general services [ 41 ]. In addition to systems which monitor energy, in a smart building, the control of water resources takes a key role by monitoring consumption and reducing leakage. Thus, several studies—as described in the next section—have investigated the advances in devices and technologies for smart water management.
Overall, the key technical elements of a smart building are (i) building devices and solutions—i.e., technologies for energy generation and efficiency to guarantee occupants’ comfort, safety, and health; (ii) automation technologies—sensors for data collection and actuators to issue commands via control and management platforms; (iii) management and control platforms—software to collect, process and analyze information; (iv) connectivity—communication protocols, wireless or wired, that allow communication between sensors, actuators, and control and the management platform [ 5 , 40 ].
Sensors allow for consumption management, monitoring of system performance, and activation of appropriate interventions to solve equipment malfunctions and prevent alarm triggers. Through this predictive maintenance, in case of an error message, the system collects data to determine the causes and automatically adapts to any problem [ 41 ].
Typically, it is possible to identify two main categories of smart building advantages: (i) hard benefits—quantifiable in monetary terms (energy savings, productivity optimization, predictive maintenance); (ii) soft benefits—linked to the improvement of habitation socio-environmental conditions (environmental sustainability, safety, comfort, remote management, remote control, interoperability) [ 42 ].
Finally, according to [ 43 ], smart buildings have five fundamental features: (1) automation—i.e., the ability to accommodate automatic devices or perform automatic functions (remote detection and control of environmental parameters, remote activation, and switching off of devices); (2) multi-functionality—i.e., the ability to allow the performance of more than one function in a building; (3) interactivity—i.e., the ability to allow interaction among users; (4) adaptability—i.e., the capability to learn, predict, and satisfy the needs of users and mitigate the stresses from the external environment; and (5) efficiency—i.e., the ability to save time and costs.
3. Overview of Technologies for Smart Water Management
Water is priceless and vital for every creature; it is a human need necessary for all economic operations. Regardless, the increase in the population is putting the water supply system under severe strain. In this context, smart water management carried out with the application of sensors and telemetry for communication and measurement can be a valuable solution to improve the efficiency of water distribution systems [ 35 , 44 , 45 ].
The sensors enable real-time monitoring of hydraulic data, automatic monitoring, and alerts from the Cloud platform in case of events such as water leakage or overuse. Smart water systems’ main utility are remote control of valves and pumps [ 45 ], which can measure pressure, flow, and consumption [ 17 ]. Smart sensors benefit from new intelligent management systems allowed by digital technologies to provide more sustainable and resource-efficient solutions.
Overall, it is possible to include leak detection and leak localization for water consumption monitoring, specifically water loss management, by using noise sensors and accelerometers, popularly used in water distribution infrastructures [ 17 , 46 ]. While regarding pressure measurement, it is possible to use IoT technologies, such as electromagnetic and ultrasonic flow meters and sensors [ 44 , 47 ].
Moreover, the necessity of analyzing vast amounts of data while improving the entire efficiency of systems has directed the attention of the water sector towards advanced digital tools, such as operational digital twins [ 48 ]. Digital twins can be realized as combinations of models and real-time data that could present a digital representation of a specific sector in a water system.
A proper water management system optimizes the use of the resource by reducing waste and ensuring sustainable supply. Improving devices and technologies to obtain this purpose is a crucial aspect of preserving water. Thus, it seems that smart water management has to start from the building scale.
Therefore, based on this background, this section presents an overview of the available devices and technologies for smart water resource management, primarily at the building scale.
To carry out this review, Google Scholar, Scopus, Web of Science, and IEE Xplore were considered as research repositories for the identification stage. The first qualification criteria included the following potential keywords and their combination (search strings): “smart sensors”, “smart devices”, “smart water”, “smart building”, “smart home”, “Internet of Things”, “water management”, “water monitoring”, “water level”, “smart tank”, “ultrasonic sensor”, “water flow sensor”, “smart water meter”, “smart monitoring”, “water demand”, “leak detection”, and “pipeline leakage”.
Starting from this first selection criteria, the search strategy can be shortly described as follows: (i) use of the previously defined keyword strings in various databases; (ii) selection of only published English papers in scientific peer-reviewed journals and conference proceedings in the last 30 years (1992–2022); (iii) preferring technical papers over surveys or other review articles; (iv) review of a sufficient description of devices and technologies; (v) detailed analysis of each device and technology and final inclusion in the overview.
Based on this search strategy, the overview was organized into three main sub-sections: water level, water consumption, and leak detection. Finally, 61 publications were selected (34 journal articles and 27 conference papers), published from 1992 to 2022, as reported in Figure 1 , while the frequencies of the keywords in the selected publications are shown in Figure 2 .

Number of selected publications per year (1992–2022).

Graphical representation of the more frequent keywords in the selected publications.
3.1. Water Level
Monitoring resource consumption plays a crucial role in intelligent water management. Overall, this is possible by installing water level measuring devices in a water tank or similar storage, whether in private or commercial applications. These measure devices generally consist of an emitter that generates the ultrasonic signal and a receiver element that receives the reflected wave [ 1 ]. The distance between the sensor position and the water surface is proportional to the delay between emission and reception of the ultrasonic wave packet [ 49 ].
In this regard, Min-Allah et al. [ 50 ] have developed an Android application based on the Internet of Things (IoT) for monitoring tanks’ water levels. The system works by using an ultrasonic sensor attached to the water tank that keeps track of the water level and which triggers an alert for the user if the water level is below a threshold level or empty. The architecture of this smart monitoring of water tanks system is divided into three main layers: (1) the physical layer (nodes and communication technologies that collect data and send them to the service layer); (2) the service layer (application/business logic, various tools for data analytics); (3) and presentation layer (visualizes the information to the user and allows user to interact with the system). In this proposed system, the physical layer is the physical environment (water source and associated ultrasonic sensor, which uploads data to the cloud server). The data can then be visualized via an Android application, which provides several widgets that update in real-time as soon as the ESP8266 Wi-Fi/Firebase is updated with the data stream.
While in the study [ 49 ], a tank height of 30 cm with an automatic motor to avoid water wastage is considered. If the water level becomes less than 20% of the tank height, the microcontroller sends a signal to the relay to turn on, and the motor starts. Instead, if the water level is greater than or equal to 80%, the relay automatically turns off and the engine also turns off. A message is sent to the user in both situations: in the first case, “Alert: level is 20%”, in the second case “, Alert: level is 80%”.
Raspberry technologies were also used by Sivaiah et al. [ 51 ]. The study suggests an IoT-based water monitoring system using an ultrasonic sensor. The measured level data are sent to the cloud thanks to Raspberry pi’s built-in Wi-Fi, and data readings are taken in real-time (every 20 s). The user can monitor the devices directly from the cell phone dashboard, viewing the water level.
Perumal et al. [ 52 ] proposed an IoT-based water monitoring system that measures the water level in real-time, applying IEEE802.11 communication standards and integrating a wireless gateway within the consumer network. The server collects the water monitoring data forwarded by the gateway, stores them in a database for analytics, and displays them in a web-based dashboard.
Dutta et al. [ 53 ] have presented a prototype of a smart building using technologies such as IoT, fog, and cloud. The idea is to measure the distance of the water level’s upper layer from the sensor attached point. When the tank water level becomes less than a threshold value, sensor HCSR04 automatically triggers the pump switch, sending water to the tank. After reaching the maximum level (threshold), the switch is turned off automatically. Using a fog server reduces internet traffic and makes the system more agile and responsive.
Shah et al. [ 54 ] have investigated a water level monitoring and control system with IoT and Android applications. Microcontroller ESP8266 obtains the maximum and minimum level values through the Firebase cloud. Ultrasonic sensors are used, and based on the water levels, the motor status is controlled by the android application, which shows the percentage of current water status instantaneous value. The use of ESP and an ultrasonic sensor have reduced cost-effectively and made the proposed project economical.
Praveen et al. [ 55 ] have addressed the household water overflow problem. The primary objective of the proposed model is to monitor the water tank level and sump visually. This research utilizes an ultrasonic sensor which is connected to an ESP module. The entire code is integrated with an Arduino IDE, and the real-time flow is displayed graphically via Adafruit. The proposed system helps the users to save water and understand their consumption rate.
In another study, Khan et al. [ 56 ] have shown a solution for water shortages in Saudi Arabia by developing a water monitoring system for customers. The prototype consists of two parts: the first is a website to display statistical information, enabling decision makers to implement more efficient water distribution policies and for customers to monitor their consumption rate and alert them to leaks. The second part consists of the hardware that detects the level and sends readings to the server for presentation on the website. The system has an ultrasonic sensor installed in the water container, to which a microcontroller is connected.
While in [ 57 ], a system was developed to monitor water use, prevent overflow, and find ways to save water. An ultrasonic sensor (HCSR04) measures reservoir level, while a flow sensor (YF-S201) measures water flow rate and total consumed volume. The level, flow rate and volume data are sent from the node MCU to the mobile application using the message queuing telemetry transport protocol (MQTT). Node MCU has been programmed by using Arduino IDE. Moreover, it is possible to control the solenoid valve operating as a tap from the mobile application.
Veselinović et al. [ 58 ] developed a system to save water in the toilet tank based on an ATMega 328 microcontroller. An ultrasonic sensor, water overflow sensor, and flush tank sensor are used for providing information to the microcontroller. The authors propose to add a Wi-Fi module and implement wireless communication between the device and user application as future progress.
In another study [ 59 ], the authors proposed a water-saving tool that automatically turns the water tap on or off using Arduino Uno with an ultrasonic sensor (HC-SRF04) and fuzzy logic algorithm to make the decision. The findings obtained during the experiment showed that water saving could be up to 70% per day, with beneficial economic effects for users and water providers.
Khan et al. [ 60 ] have presented an intelligent water level measurement using an Arduino Mega 2560 microcontroller, an ultrasonic sensor to detect the water tank level, a flow-meter, a Wi-Fi module, a display, and a pumping unit. The system saves inadequate water and electrical consumption by turning the pump unit on or off based on the water level.
Olisa et al. [ 61 ] have developed a system to monitor water quality and level in a two-tank water system. A pulse-echo ultrasonic technique (HCSR04 ultrasonic sensors) was used to control the water level. Other system components are a microcontroller (ESP32 with an integrated Wi-Fi module and Bluetooth module for wireless communication), actuator, electronic control valves, water pump, turbidity sensor, and pH sensor. With an Android mobile application, the user can monitor the level and quality of water in the overhead tank.
Table 1 presents a summary of the main components considered in the investigated systems for smart water level monitoring.
Summary of the main components used in the investigated smart water level monitoring systems.
3.2. Water Consumption
In recent years, automated, real-time monitoring systems have been developed for intelligent management of water consumption and properly quantifying it. One method to manage consumption involves smart water meters, based on the Internet of Things (IoT) and cloud computing, equipped with specific algorithms to distinguish between normal and excessive water use. This raises users’ awareness of water use and promotes sustainable water management.
The system proposed by Khan et al. [ 56 ], whose components have been discussed in Section 3.3 , allows homeowners to monitor their daily usage by providing illustrations for each water tap separately, as well as notifying the homeowner of a leakage possibility. In the system, all sensors are connected to a microcontroller to estimate consumption rate, leak detection, and tank level sent through the Ethernet shield to the website. This system can reduce shortages by driving water pumping to the most needed districts and reducing water waste since around 40% of water can be stored.
Santos et al. [ 62 ] have developed an android application to monitor power and water volume data that tenants and landlords can view. The meter devices read and measure the values during water and energy consumption, then process and combine the equivalent values. Thus, the data—stored in memory—is sent to the users’ smartphones to display the consumption. From the evaluations, the device is reliable, accurate, functional, and user-friendly.
In the study [ 63 ], a smart water meter system is developed, where the flow sensor reading is noted by NodeMCU and transmitted to the ThingSpeak Cloud platform. Machine learning is used to identify whether the flow pattern is normal, excessive, or continuous. When excess water flow occurs, the systems generate alerts sent to the users by email.
Vrsalovic et al. [ 64 ] have presented the development of a smart water meter IoT architecture. Axioma Qalcosonic water metering devices, equipped with LoRaWAN radio technology, measure the water consumption of some university building blocks. By observing the graphical representation of the average hourly water consumption, it is possible to detect uneven consumption that can indicate the presence of leaks in the system.
Suresh et al. [ 65 ] have proposed an innovative approach to perform automated water-meter reading and update consumption information. This approach differs from existing commercial methodologies, because it uses low-cost IoT hardware and a smartphone application. The approach was evaluated on a small section of the water distribution network of the Institute campus, saving around 14% of water thanks to corrective actions, replacements, and operations.
In another study, Tamilselvan et al. [ 66 ] developed a method to improve regular water delivery and control it from a central server to reduce water supply problems. The system uses an Arduino as a minicomputer, water flow sensor, and solenoid valves.
A wireless water consumption monitoring system whose main components are water flow rate/temperature sensors to collect data, which are routed to a remote computer server by home Wi-Fi and Internet, was designed by [ 67 ].
De Paula et al. [ 68 ] have proposed a smart system for remote monitoring of water consumption, detecting leakages and interruptions in water distribution. The hydraulic tier consists of two transducers (the water flow sensor and the pressure sensor) and one solenoid valve if some problems occur in the system. Specifically, the water flow transducer is a hall effect sensor with a digital output which can detect water flow between 1 and 30 L/min, with a maximum pressure of 1.75 MPa; by counting the number of pulses per minute, it is possible to obtain the measurement of flow and water consumption. At the same time, the pressure sensor can measure pressures in the range of 0 to 1.2 MPa. The electronic tier consists of two microcontrollers (MCUs): the MSP430G2553 MCU processes the acquired data and sends them by a UART channel to the second MCU (TI CC2650), which transfers the information to the In. IoT middleware uses a border router with Internet connectivity.
In another study [ 69 ], the FIKWater dataset is presented. The system consists of an ultrasonic flow meter (TUF2000M) installed in the main entrance pipe; the recorded data are sent by Modbus protocol to a local gateway, and then every minute data are uploaded by a standard HTTPS protocol to an online database server; finally, a CSV file with the daily readings is uploaded to a shared folder. The ultrasonic flow meter measures: instantaneous flow rate, liquid velocity, sound velocity, positive and negative accumulators, and totals (day, month, and year). The dataset contains time series of both hot- and cold-water demand data from three restaurant kitchens in Portugal, collected for consecutive periods between two and four weeks.
The study [ 70 ] presents a water management system based on wireless sensor networks (WSNs), consisting of three major components: the meter interface node, the gateway device, and the back-end system. With a web-based interface, it shows real-time and historical water consumption data. Therefore, the monitoring system can help users to reduce water consumption and identify possible leakage.
The study [ 71 ] proposed a fuzzy clustering algorithm to analyze residential water consumption patterns using smart meters.
In order to support decisions at both household and utility levels, Savica et al. [ 72 ] have created the iWIDGET system, which analyses the usage pattern of individual households, offering suggestions on how to reduce usage and take advantage of current tariff schemes, as well as sending an alert if local leakage is suspected. Therefore, by ICT, the system supports integrated water management, improving water use efficiency, reducing wastage by households, and enabling utilities to manage household water demand better.
Finally, another interesting study—although there are not many details about the IoT system—was carried out by Sodkomkham et al. [ 73 ]. It presents an integrated lean management, IoT, and MFCA system for achieving optimal water management in industrial production in Thailand. Water sensors to detect the amount and quality of water are used, but there are not many details about the other hardware and software components. In the case study, inputs, processes, and outputs were analyzed to reduce water use by recycling wastewater in processes and by applying the 3R (reduce, reuse, recycle) method. The results demonstrated a reduction in water use in production processes by 15% per year.
Table 2 shows the main components considered in the analyzed smart water consumption monitoring systems, excepting those of studies [ 71 , 72 , 73 ], since few details are reported, and study [ 56 ] since it was already shown in Table 1 .
Summary of the main components used in the investigated smart water consumption monitoring systems.
3.3. Leakage Detection
Water losses frequently occur in the city water distribution network [ 74 ]. Thus, leakage prevention and break identification are crucial to limiting water wastage. In this regard, to prevent water losses and decrease public risks, many techniques have been proposed with diverse applications for detecting the occurrence and sizes of leakage in water pipeline systems [ 75 ]. These devices—often connected to the network—can transmit information in real-time, control all of the data, and detect irregular water consumption and alarms related to leaks and micro leaks. Through these technologies, the whole building’s entire water system is effectively managed, with the possibility—for example—of water remotely shutting off or automatically shutting off in case of leaks to limit damage from uncontrolled water spillage into the building.
Thus far, several leak detection methods have been presented, and as reported in [ 76 ], they can be classified into three main categories: (i) biological methods—i.e., conventional leak detection methods based on individuals’ experiences; (ii) software-based methods—which use different computer software to detect leaks in a pipeline; (iii) hardware-based methods—which consider the use of different hardware devices to detect and locate the leak. The software-based method can be distinguished into flow or pressure change, mass or volume balance, dynamic model-based system, and pressure point analysis. The hardware-based methods can be classified into four types according to the principles of devices: visual devices, acoustic devices, gas sampling devices, and pressure wave detectors.
Based on this main classification, several studies that proposed software-based methods were found in the literature [ 77 , 78 , 79 , 80 , 81 , 82 , 83 , 84 , 85 , 86 ]. While volume balance method, as reported in [ 87 ], is one of the earliest computer methods developed. Moreover, as discussed in [ 88 ], for the continuous monitoring of a pipeline, another method is ATMOS PIPE which is a statistical pipeline leak detection technique. In another study, Salam et al. [ 89 ] developed a computerized online system to detect leakage with pressure analysis obtained from the EPANET software using the radial basis function neural network method.
Similarly, in the literature, several hardware-based methods were found. The majority of the studies have considered acoustic methods or methods based on vibration [ 90 , 91 , 92 , 93 , 94 , 95 , 96 , 97 , 98 , 99 , 100 , 101 , 102 , 103 , 104 ]. The studies [ 76 , 88 ] have considered visual observation methods, while ground-penetrating radar (GPR) methods were used in [ 105 ].
The researchers have shown a strong interest in vibration techniques for leak detection, considering accelerometers or ultrasound to measure the vibration signal of the water pipe [ 103 ]. In this regard, ref. [ 98 ] proposed a model for a real-time monitoring system based on wireless accelerometers. The accelerometers are installed on the exterior of the pipelines. Analysis of the vibration signal of each accelerometer sensor was assessed to determine the monitoring index. The collected data from the investigation were evaluated using the support vector machines (SVM) technique. A leak threshold was determined such that if the signal increased above the threshold, a leak status was identified. Experiments were performed on one-inch cast iron pipelines and one-inch and two-inch PVC pipelines using single event leaks, and the results were displayed. The developed models showed promising results with 98.25% accuracy in distinguishing between leak and non-leak states.
Almeida et al. [ 100 ] have investigated the combined filtering effects of the sensors (hydrophones, geophones, and accelerometers) and pipes for leak detection. The results showed that all three sensors could detect and locate a strong leak; on the other hand, a weak leak was not detected by the hydrophone sensors since there was noise in a narrow range of low frequencies—not related to the leak—that dominated the signals.
Study [ 103 ] proposed a non-attached ultrasound PVC pipe monitoring system. The system, which works with V-type ultrasonic air-coupled ultrasonic transducers, was tested in the laboratory. A pair of MA40S4R emitting ultrasound toward the pipeline were considered. The laboratory tests showed efficiency in recognizing leakages up to 0.4 mm in diameter with an accuracy of 94.97%, even for water pressure (less than 1 bar).
Dewi et al. [ 91 ] show the difference between output data from the normal pipe and pipe with leakage: the normal pipe produces more vibration than the pipe with leakage.
While in the study [ 106 ], a new interpretation of the cross-correlation process for estimating time delay in a vibroacoustic system is presented. Test data recorded from a specially constructed leak-detection facility located at the National Research Council in Canada were considered for validation. The results showed little difference in determining the time delay between the leak signals in the time domain using cross-correlation (GCC) methods or in the frequency domain using generalized phase spectrum (GPS) methods.
An interesting application is also the indirect approach to the problem, accomplished through pressure and temperature measurement and monitoring, which can help locate leaks. In this regard, Sadeghioon et al. [ 107 ] have designed and constructed a sensor for real-time leakage detection in pipelines based on measuring indirect relative pressure and temperature changes in plastic pipes. During the measurement campaign, the relative pressure and temperature sensors recorded leak tests and daily pressure changes.
A microelectromechanical systems (MEMS)-based wireless sensor network was developed by [ 93 ]. The system incorporates MEMS accelerometers for measuring flow-induced vibration on the surface of a pipe to evaluate the water pressure change due to leakage. Therefore, this study proposed using accelerometers attached to the pipe’s external surface instead of pressure sensors, whose installation is generally invasive. Thereby, this method presented a low-cost, nonintrusive pipeline monitoring system.
Daadoo et al. [ 108 ] focused on wireless sensor network applications for leakage detection in underground water pipelines. The wireless network system uses wireless mobile sensors that can detect breaks and save energy, time, and cost by smart water leakage detection (SWLD) in pipelines, measuring tank water level and controlling the pump to activate it when the water level is low.
Almazyad et al. [ 109 ] explored the use of mobile sensors by showing a simulation of a water pipeline monitoring system for leakage detection using radio frequency identification (RFID) and wireless sensor networks (WSNs); the system is for long-distance surface water pipelines.
The study [ 110 ] presents the development of a water pipeline monitoring system over ZigBee networks. The system’s main components are 6DOF MPU6050 sensors to collect the vibration data, Arduino UNO controller board, XBee module, and decision support system. The data are analyzed offline to define the conditions of the water pipeline.
Generally, the methods for leakage detection are either expensive, unscalable, or feasible only in the short term. Thus, Hester et al. [ 104 ] presented using environmentally powered embedded adaptive sensors to provide cost-effective water-monitoring infrastructure.
Most studies have analyzed monitoring systems for leakage detection for long-range pipes, while [ 90 ] presented a method of monitoring the condition of domestic pipelines. The system considered the operation of acoustic sensors and a couple of transducers (a transmitter and receiver). This approach is cheap and easy to install and maintain for homes and most other public facilities.
Britton et al. [ 111 ] have provided smart metering technology for households, allowing them access to water loss information. The residential leakage communication strategy has reduced minimum nighttime flows by a significant 89% throughout the study, while the group that received no communication increased consumption by 52%.
Kawarkhe et al. [ 49 ] designed a leakage detection system for pipes using two flow sensors. If the flow measured by the first sensor and the second one is not equal, a leakage is detected; thus, the GSM sends the message to the user.
Veselinovic et al. [ 58 ] have considered EPA data on average household leaks and the most common types of leaks. This study developed a smart home system to save water in the toilet tank, using a controller that opens and closes the valve and detects water leaks and toilet overflow. When a leakage or overflow is detected, the device performs the corresponding operation and indicates the type of error by turning on the specific LED (light-emitting diode). This paper explains water level measurement, leak detection, as well as the implementation of the device.
De Paula et al. [ 68 ] have proposed a solution in which all smart devices can communicate with each other, making everyone aware of the leakage problem. To perform the measurements, they have proposed to use two transducers (a water flow sensor and a pressure sensor) and a solenoid valve to stop the leaks as soon as they are detected. In a building, the water flow transducer must be installed near to the building water box, while the pressure transducer must be near to the hydraulic devices (shower, washing machine, or tap). Thus, if the system detects any abnormality, a relay activates the solenoid valve, and the water flow to the hydraulic devices is stopped.
As it emerged from the analysis of the selected studies, several leakage identification applications were performed for large-scale systems (urban drainage networks); some have presented monitoring system prototypes that can be used for different scales, while others—as already discussed in the previous sections—have analyzed the leakage detection in the building. Given the wide range of applications in this field and the different discussed methods, in Table 3 , the main components of the system for leakage detection of 10 selected studies from those that have considered hardware-based methods are reported.
Summary of the main components used in some hardware-based investigated systems for smart leakage detection.
4. Smart Water Harvesting Systems
Increasing urbanization and climate change have impacted the natural water cycle with considerable effects in terms of increased runoff and flood hazards [ 112 , 113 ] and water scarcity phenomena [ 114 , 115 ]. These environmental impacts encourage widespread sustainable technologies, known as low-impact development systems (LID)—engineering techniques widely investigated in literature for their beneficial effects in reducing environmental impacts and obtaining proper water management in urban areas [ 116 , 117 , 118 , 119 , 120 ].
In this context, a promising strategy to save water and optimize its management is the implementation of water harvesting systems. These systems collect and reuse water from multiple sources (rainwater, greywater, atmospheric water). Thus, based on the type of water collected, these systems can be classified into rainwater harvesting systems and grey water harvesting systems.
The rainwater harvesting (RWH) system provides several benefits at different scales; it supplies decentralized water, manages stormwater, and increases local water security. This system collects rainwater from roofs, rooftop terraces, and impermeable surfaces to be reused on-site for different purposes, including irrigation of green roofs and gardens, flushing toilets, etc. Several studies have investigated the efficiency of these systems for water-saving and runoff mitigation [ 115 , 121 ].
Greywater is the wastewater collected from domestic washing operations—including showers, bathtubs, kitchen sinks, dishwashers, and washing machines; excluding blackwater sources (toilet, bidets, and urinals). Given its amount, this source can represent the most significant potential source of water savings for domestic use. Of course, given its low quality, treated greywater is mainly utilized for toilet flushing, garden watering, and car washing [ 122 , 123 ].
Although the conventional RWH systems are widespread, the advances in IoT technologies have affected their utilization, posing new optimization challenges. Thus, in the literature, smart water harvesting systems are gaining popularity.
In this regard, Maer et al. [ 124 ] proposed an original approach to classical reusable water collecting and reuse systems—i.e., rainwater harvesting systems (RHWs), drain water collecting, and wells—by automating the system using smart home technology. A reusable hybrid system combines available water sources at a specific location or time. This study emphasizes the most efficient and cost-effective solution to be implemented. The designated area of study is a household in Cluj-Napoca, Romania. The proposed water catchment and management system model was designed to be integrated, monitored, and controlled via smart home technology to use PV panels and low-consumption equipment. A proprietary web interface is used for automatic control and supervision. The authors used original approaches to reducing water scarcity by integrating environmentally friendly hardware equipment. This research aimed to provide an integrated smart home, tested, and functional solution for one of humanity’s biggest challenges—water scarcity.
Oberscher et al. [ 125 ] presented an innovative smart rain barrel integrated into a pilot project for smart cities, where weather forecasts and time-controlled filling levels of different low impact development (LID) structures and the connected sewer system are used for real-time control (RTC).
In another study, Behzadian et al. [ 126 ] investigated a smart RWH system that proactively controls the tank water level to guarantee sufficient storage to receive stormwater runoff. The analysis using the WaterMet2 model emerges that the smart system can significantly mitigate local flooding and supply harvested rainwater to non-potable residential water consumption.
In conclusion, a smart combination of sustainable systems and intelligent technologies can significantly improve water resource management and enhance sustainable development.
5. Discussion, Current Challenges, and Future Directions
Section 3 provided an overview of IoT-based smart water monitoring and control solutions, focusing primarily on systems that are useful at the building scale and then at the urban scale. From this overview, it emerges that the crucial components of an IoT-based water management system can be principally classified into hardware units and software units. As reported in [ 12 ], sensors, actuators, and smart meter devices—main hardware components—are used to collect data, and communication technology is used to connect the overall elements of the systems; while the cloud platform stores, processes, and analyses the data.
Based on this main classification, ultrasonic sensors are the most used in analyzing the studies on the systems developed to monitor water levels. This type of sensor generates ultrasonic sound waves bombarded on the water’s surface [ 54 ]. Among these devices, HCSR04—consisting of an ultrasonic transmitter, receiver, and control circuit—is commonly used for non-contact distance measurement from 2 cm to 400 cm [ 51 ]. Another sensor used in systems to monitor water consumption is the Hall-effect-based water flow sensor. Among these devices, the YF-S201 is one of the most used. This type of sensor has a pinwheel sensor to measure the amount of water moving through it, and it also has an integrated magnetic Hall-effect sensor that outputs an electrical pulse with every revolution [ 63 ]. As reported in [ 57 ], this sensor has an operating flow rate of 1 to 30 liters per minute. Smart water metering devices are widespread in the water consumption monitoring system. Finally, by analyzing the developed systems for leakage detection, accelerometers were the most used. In this regard, accelerometers have garnered researchers’ attention since they can be used as a complete leak detection system to detect vibration signals emitted by leaks [ 127 ].
Moreover, as reported in [ 15 ], the use of wireless sensor networks—considered in different studies of this overview—cannot be overlooked.
The control unit is generally the core hardware of all monitoring and control systems; from the review conducted here, the ESP Wi-Fi modules, Raspberry Pi, and Arduino in their different categories resulted in the most used. More in detail, ESP 8266 is a Wi-Fi module used as a microcontroller programmed to implement logic statements [ 54 ]. It comes with a powerful Wi-Fi module, which allows information transfer through Wi-Fi and consists of a storage capacity and powerful enough onboard mechanism to coordinate with the sensors [ 55 ]. It is cheaper than Raspberry Pi and other micro-controllers, making the system more affordable. Moreover, this microcontroller with specific sensors effectively reduces cost, providing flexible, economical, and easy configurable systems [ 57 ]. Raspberry Pi is configured as a credit-card-sized microcomputer based on a Raspbian Linux operating system with less complexity and more affordable solutions for wireless monitoring [ 128 ]. However, several of the investigated studies have used Arduino boards as a control unit and, more in detail, Arduino Uno [ 59 , 104 ], Arduino Pro [ 53 ], Arduino Mega2560 [ 60 , 108 ], and Arduino Ethernet SHIELD [ 66 ]. As reported in [ 129 ], Arduino Uno, which is based on ATmega328 microcontroller; and Arduino Pro, which can be based on ATmega168 or ATmega32, belong to the Arduino Boards category classified as Entry Level Board; while Arduino Mega 2560, which is based on ATmega2560, belongs the category of enhanced features boards. Entry level boards are most easy to use and program, while enhanced features boards are designed for complex project development and present more features and performance than entry-level boards. Finally, Arduino Ethernet Shield (Internet of Things (IoT)) facilities connectivity to Arduino board. Generally, all the Arduino Shields are specially designed for beginners to overcome the complexity of connecting components and to add more hardware resources. Arduino is widespread primarily due to its features: an independent platform, low cost compared to other microcontrollers, open source hardware and open source software, and ease of programming via Arduino IDE.
As it is possible to detect in Section 3 , different communication technologies have been considered (Wi-Fi, Bluetooth, RFID, Zigbee, LoraWAn, Cellular network, etc.). Bluetooth and RFID (radio frequency identification) are wireless connectivity technologies with a short-distance communication range (at most 10 m); ZigBee and Wi-Fi operate within a medium distance range (10 to 100 m); while cellular networks (2G/3G/4G/5G), and low-power wide-area (LPWA) technologies (which includes LoRa) operate in the long-distance communication range category [ 130 ]. Among these technologies, ZigBee is a wireless communication technology operating on the IEE 802.15.4, which addresses the need for low-rate, low-power, and low-cost wireless networking [ 131 ]. In this regard, as reported in [ 132 ], it presents a low rate between 20 kbps and 250 kbps, compared to Wi-Fi’s 11 Mbps or Bluetooth’s 1 Mbps; network join times of around 30 ms, compared to Wi-Fi’s up to 3 s or Bluetooth’s up to 10 s; lower power consumption (ZigBee devices can operate for several years on a single battery); low cost of products and cheap implementation. Moreover, it can support hundreds of devices compared to Wi-Fi which can support up to 32 devices or Bluetooth which can support up to 7. Finally, LoRaWAN (LoRa wide area network) is a network based on LoRa technology, which is one of the most promising low-power wide-area communication technologies. It enables long-range transmission with low power consumption, and it can achieve data rates between 0.3 kbps and 27 kbps. How to implement a flexible LoRa network with an effective cost is still an open challenge [ 131 ].
Another crucial element in IoT-based systems is the cloud platform, which—together with big data platforms—is suitable for storing large-scale datasets through database management systems, preprocessing, statistical analysis, and data visualization [ 11 ]. These platforms perform logical analysis and complete control over the functionality of the IoT-enabled devices [ 13 ]. Some of these also provide apps that monitor and control the IoT elements by mobile devices [ 10 ]. Following the widespread of IoT devices, several IoT cloud platforms were developed, such as ThingSpeak, Blynk, Arduino Cloud IoT, IBM IoT, Adafruit, and others [ 13 ]; some of these were also considered in the manuscripts investigated in Section 3 . In more detail, ThingSpeak Cloud Platform is an open IoT data platform that can easily configure devices to send data through standard IoT protocols and visualize the sensor data in real-time [ 63 ]. ThingSpeak IoT Platform and Arduino IoT Cloud allow data collection in private channels with free hosting for channels, app integration, and event scheduling [ 133 ]. The IBM Watson IoT Platform can be used for any IoT solution, including smart homes; it provides machine-learning services to adopt into IoT applications and data analytics [ 134 ], while Adafruit provides different statistical tools on single clicks [ 55 ]. Finally, as reported in [ 134 ], different Cloud infrastructures have been developed (OpenStack, OpenNebula, and CloudStack) to monitor, manage, and offer an infrastructure to deploy Cloud platforms. More in detail, OpenStack consists of a pluggable set of components, while the other two present a centralized architecture; therefore, OpenStack better meets the user’s needs but requires higher installation efforts than the others. To address communication requirements and scalability issues, Merlino et al. [ 135 ] proposed Stack4Things, an extension of the OpenStack platform to enable a cloud-oriented infrastructure for IoT management.
In Section 4 , the use of smart technologies to optimize the use of sustainable solutions for water harvesting systems is analyzed. These studies show the enhanced efficiency of smartly equipped conventional harvesting systems.
Therefore, this overview identified the main components of the IoT monitoring and control of smart water management systems, and a comparison was also presented. However, through in-depth analysis, each technology presents advantages and limitations that should be overcome to increase the applicability of these systems starting from the building scale.
Cost of deployment, power usage, maintenance, privacy and security, connectivity coverage, complexity, and ease of operation are still open challenges. In this regard, the future development of low-cost devices with higher energy efficiency is crucial in supporting and driving widespread IoT applications in the water management sector. According to Khanh et al. [ 131 ], another aspect that will continue to be a research topic for academic and industry research in the future is privacy and security. As reported in [ 14 ], the amount of data collected in IoT-based systems—like those investigated in this review—is vast and vulnerable to cyber threats, especially during the transmission to the data server. Moreover, as previously discussed, the commonly used wireless communication protocol has some limitations concerning the power requirement of some technologies, as well as the communication distance they can cover [ 136 ].
Moreover, another major challenge is the perceived complexity of smart water systems related to installation, operation, and maintenance activities [ 63 ]—as well as the need to develop smart water management systems to be more adaptable and replicable in different contexts and locations [ 11 ].
In conclusion, based on all of this discussion, an in-depth analysis of the investigated articles supports the readers in identifying the main challenges, relevant recommendations, and future directions for IoT applications for smart water management.
6. Conclusions
Increased population and industrial activities combined with climate change present a serious issue regarding water resource availability. The water scarcity phenomenon is increasing and represents one of the global environmental impacts. Thus, monitoring water usage and adequately managing this limited resource is one of the main aims of researchers in recent years. Proper water management can optimize the use of this resource by reducing waste and managing supply. Several studies have investigated the main technologies to reduce water wastage. Most of them focus on the smart management of this resource through IoT advanced technologies.
This paper presents an overview of the innovative systems for smart water resource management. Specifically, we focused on the innovative technologies to monitor, control, and manage water levels, water consumption, and water leakage starting from the building scale. Finally, innovative technologies were analyzed by combining sustainable systems—such as water harvesting systems—generally used to save and reuse water resources.
This comprehensive overview revealed the importance of investigating the potentiality of water-saving innovative and sustainable technologies to optimize resource management by limiting technical losses and human overuse.
Acknowledgments
The author S.A.P. gratefully acknowledges the financial support from the Italian Ministry of University and Research (D.M. n. 1062/2021)—REACT EU—National Operational Program on Research and Innovation (PON R&I) 2014–2020—Axis IV; Action IV.4, Action IV.6. CUP: H25F21001230004. IC: 1062_R18_GREEN.
Funding Statement
The author S.A.P. is supported by the Italian Ministry of University and Research (D.M. n. 1062/2021)—REACT EU—National Operational Program on Research and Innovation (PON R&I) 2014–2020—Axis IV; Action IV.4, Action IV.6. CUP: H25F21001230004. IC: 1062_R18_GREEN.
Author Contributions
Conceptualization S.A.P., M.M., G.S. and P.P.; methodology, S.A.P., M.T. and P.P.; formal analysis, S.A.P. and A.C.B.; investigation, S.A.P., A.C.B. and M.T.; data curation, S.A.P., A.C.B. and B.P.; writing—original draft preparation, S.A.P., A.C.B., M.T. and B.P.; writing—review and editing, S.A.P., A.C.B., M.T., E.G. and B.P.; visualization, S.A.P., M.T., B.P. and E.G.; supervision, M.M., G.S. and P.P. All authors have read and agreed to the published version of the manuscript.
Conflicts of Interest
The authors declare no conflict of interest.
Publisher’s Note: MDPI stays neutral with regard to jurisdictional claims in published maps and institutional affiliations.
Smart water management using IOT
Ieee account.
- Change Username/Password
- Update Address
Purchase Details
- Payment Options
- Order History
- View Purchased Documents
Profile Information
- Communications Preferences
- Profession and Education
- Technical Interests
- US & Canada: +1 800 678 4333
- Worldwide: +1 732 981 0060
- Contact & Support
- About IEEE Xplore
- Accessibility
- Terms of Use
- Nondiscrimination Policy
- Privacy & Opting Out of Cookies
A not-for-profit organization, IEEE is the world's largest technical professional organization dedicated to advancing technology for the benefit of humanity. © Copyright 2024 IEEE - All rights reserved. Use of this web site signifies your agreement to the terms and conditions.

News and Events

Research and Programs
Work with Us
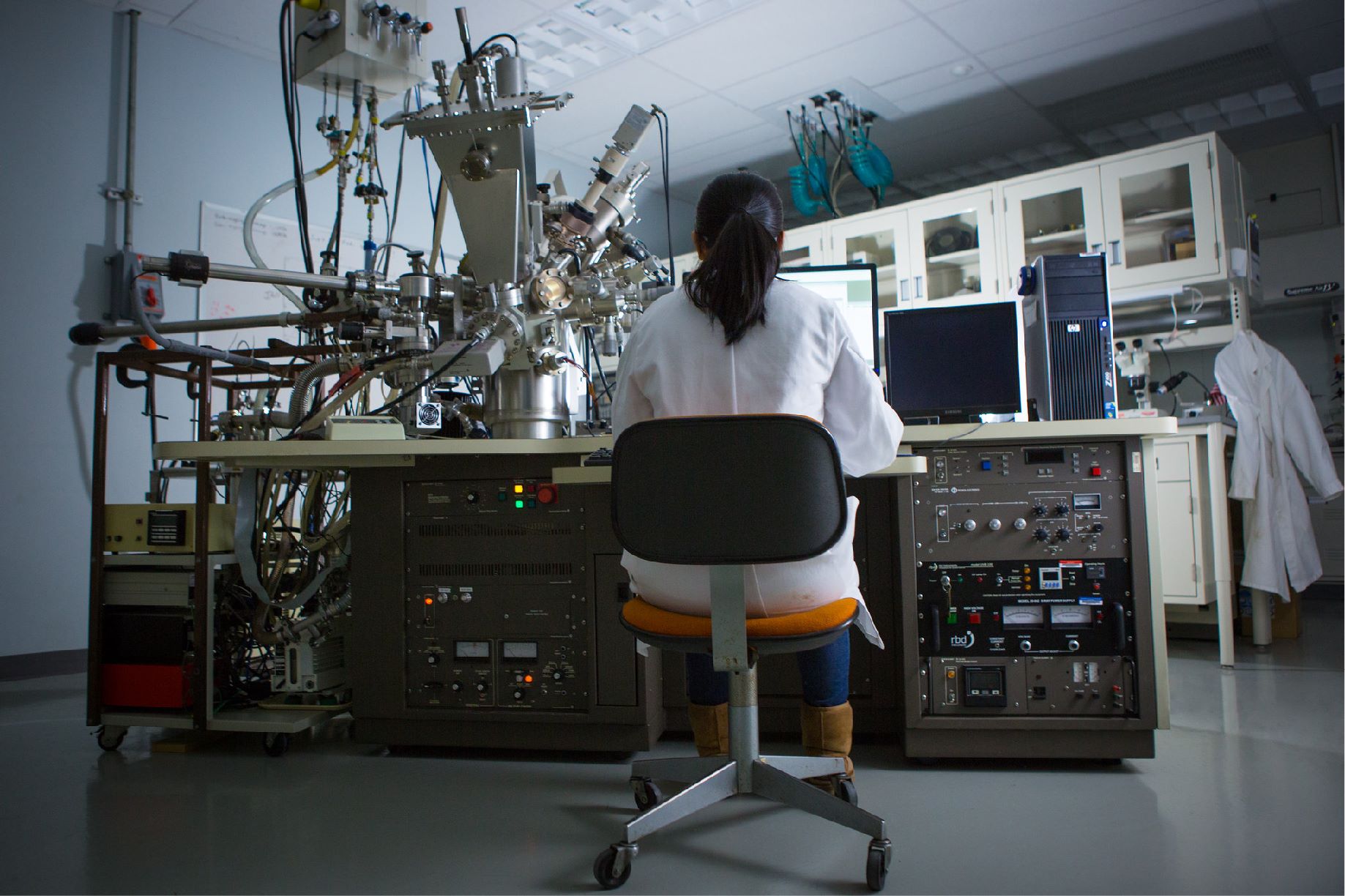
Explore our Library
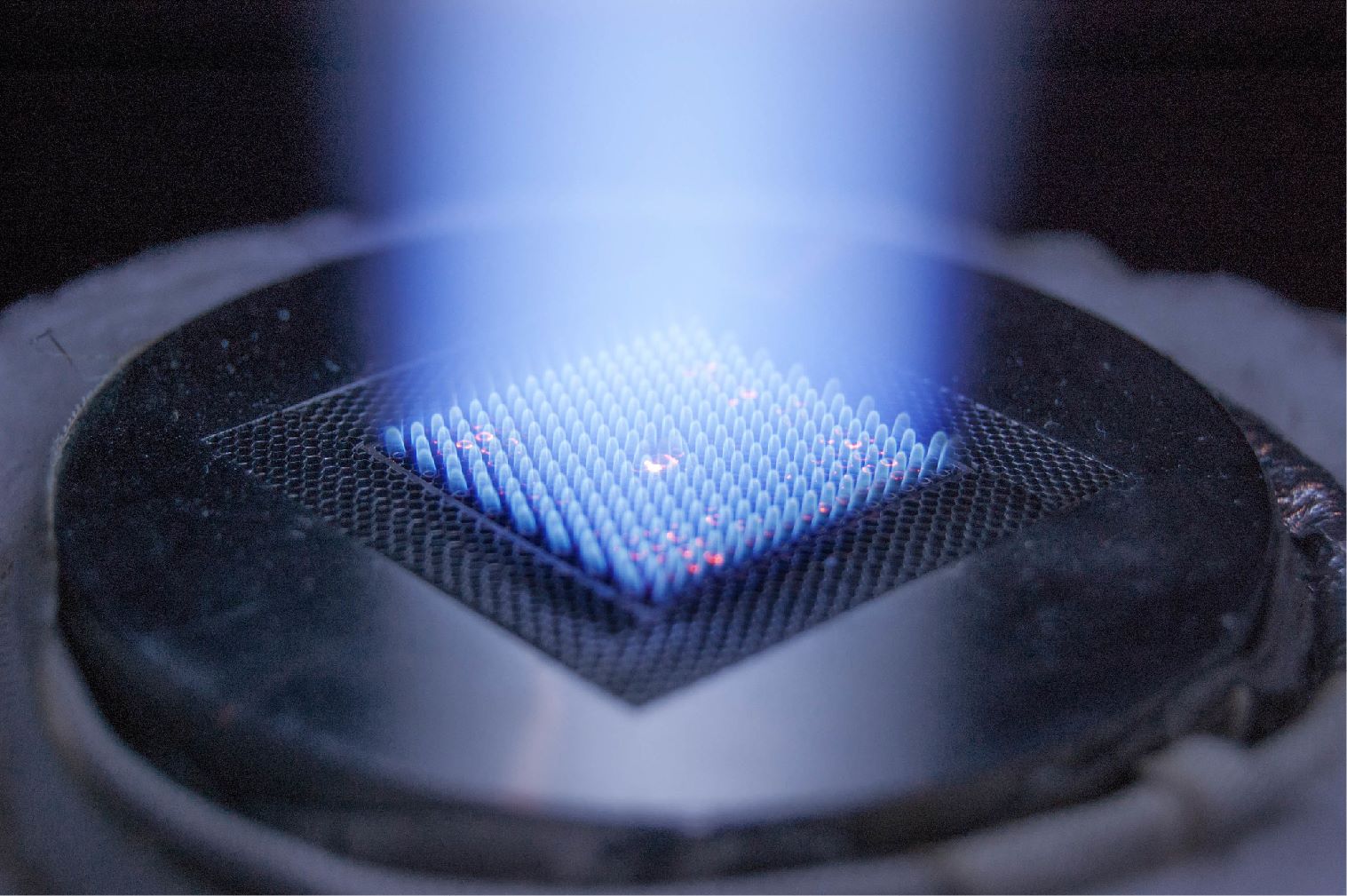
Point Source Carbon Capture
- Carbon Dioxide Removal
- Carbon Conversion
- Carbon Transport & Storage
- Hydrogen with Carbon Management
- Computational Science and Engineering
- Energy Conversion Engineering
- Geological and Environmental Systems
- Materials Engineering and Manufacturing
- Strategic Systems Analysis and Engineering
- Program Execution and Integration
- Methane Mitigation Technologies
- Minerals Sustainability
- Natural Gas Decarbonization and Hydrogen Technologies
- Advanced Remediation Technologies
- Energy Asset Transformation
- Historically Black Colleges and Universities and Minority Serving Institutions Program
- University Carbon Research Program
- Advanced Alloys Signature Center (AASC)
- Science-based Artificial Intelligence and Machine Learning Institute (SAMI)
- Center for Microwave Chemistry (CMC)
- Center for Sustainable Fuels and Chemicals (CSFC)
- Office of Energy Efficiency and Renewable Energy
- Battery Workforce Initiative
- Cybersecurity, Energy Security, and Emergency Response
- Office of Electricity
- Grid Resilience
- Direct Air Capture Center
- Net-Zero Laboratory
- Hydrogen Research & Development
- Solicitations and Funding Opportunities
- Prizes and Challenges
- Business Forms
- Site Support Electronic Reading Room
- Market Research
- Unsolicited Proposals
- Available Property
- Partnerting with NETL
- Regional Workforce Initiative
- City of Pittsburgh MOU
- Small Business Corner
- Success Stories
- Available Technologies
- Key Contacts
- Approved Categorical Exclusions
- Environmental Assessments
- Environmental Impact Statements
- Oil and Gas Projects Summaries
- NETL Fact Sheets
- NETL Newsletters
- Publication Search
- Energy Data Exchange (EDX)
- FECM External R&D Final Technical Reports
- Summary Information for External R&D Awards
- Technical Reports Series (TRS)
- Peer Review Reports
- Interagency Working Group Initial Report
- Search Energy Analysis
- Search Unit Process Library
- Mission and Overview
- Contact NETL
- Organization
- Awards and Recognition
- Site Environmental Quality
News Stories
- Conference Proceedings
- Internship Opportunities
- STEM Education and Outreach

Advancing technologies for the capture of CO 2 from point sources, such as natural gas power and industrial facilities, with minimum cost and energy penalty
The U.S. Department of Energy/National Energy Technology Laboratory’s (DOE/NETL) Point Source Carbon Capture Program is developing the next generation of advanced carbon dioxide (CO 2 ) capture concepts to support the United States in achieving ambitious goals for a greenhouse gas (GHG)-neutral economy by 2050, a carbon-pollution-free power sector by 2035, and a 50% reduction from 2005 levels in economy-wide net GHG pollution by 2030. DOE’s Office of Fossil Energy and Carbon Management (FECM) has adopted a comprehensive, multi-pronged approach for carbon management that involves the coupling of carbon capture methods (i.e., Point Source Capture (PSC) for fossil fuel-based power generation and industrial sources; and carbon dioxide removal (CDR) technologies co-located with low-carbon energy sources) with long-duration carbon storage or CO 2 utilization/conversion into long-lasting products. The PSCC Program is accelerating commercially deployable solutions that can be applied to a wide spectrum of CO 2 emissions sources, including facilities that produce power, hydrogen, ethanol, cement, or steel. Projects range from conceptual engineering and materials design at laboratory and bench scale (Technology Readiness Level [TRL] 2-5) to large-scale testing and front-end engineering and design (FEED) studies (TRL 6-7) to lower both capital and operating costs and improve the economics of PSC.
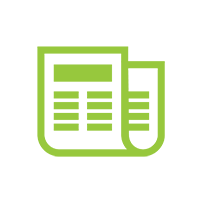
Carbon Capture Newsletter
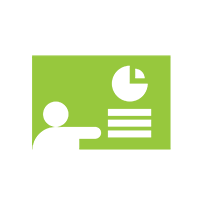
Carbon Capture Proceedings
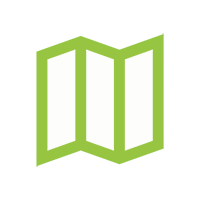
Carbon Capture Project Map
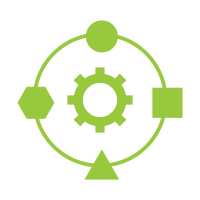
Carbon Capture Compendium
The Point Source Carbon Capture Program is advancing technologies to minimize the environmental impacts of fossil fuel-based power generation and to decarbonize existing infrastructure in the power and industrial sectors. Research and development (R&D) efforts to date have led to reductions in both capital and operating costs through implementation of energy and process efficiencies and development of advanced CO 2 capture media (e.g., solvents, sorbents, and membranes). To achieve deep decarbonization of emissions sources, the program is focused on developing highly efficient, scalable carbon capture technologies with even further cost reductions, that are capable of operation under a flexible duty cycle, and that can achieve greater than 95% carbon capture.
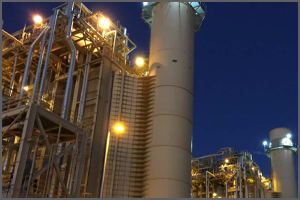
Capture from Power Generation Sources
Point source CO 2 capture in fossil fuel-based power production separates CO 2 emissions from the plant’s exhaust gas or syngas stream. The Point Source Carbon Capture Program is focused on advancing novel carbon capture materials, equipment, processes, or a combination thereof for applications in natural gas combined cycle (NGCC) power generation. R&D is underway to support testing of highly efficient component designs, material systems, and/or integrated processes and to conduct FEED studies for commercial-scale CO 2 capture at existing NGCC power plants.
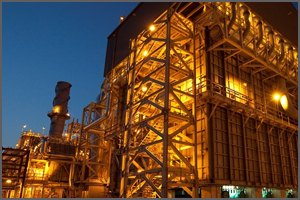
Capture from Industrial Sources
Point source CO 2 capture from industrial facilities, such as mineral production (cement and lime) plants, iron and steel manufacturing plants, hydrogen production plants, and ethanol plants—in which CO 2 emissions may be present at a higher concentration than fossil fuel-fired power plants—is a vital element in reducing CO 2 emissions. R&D is underway to test transformational capture technologies for industrial carbon capture and to conduct initial engineering design and FEED studies on commercial-scale capture systems that separate CO 2 emissions at industrial facilities.
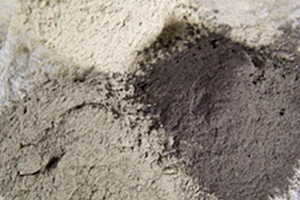
Emissions Control
Point sources of CO 2 such as power plants can result in output of byproduct residuals that can either be gainfully recycled or end up as a disposal liability. Also, various non-CO 2 emissions may be impacted as CO 2 capture retrofits are applied. R&D is underway to develop technological improvements and solutions for management, disposition, and remediation of these residuals and non-CO 2 emissions. Possibilities to increase beneficial use of residuals, to safely manage and disposition aging inactive/legacy residuals impoundments, and to use innovative technologies such as plant-based stabilization and monitoring in environmentally sound approaches, are in scope of investigation. These efforts will promote environmental justice and help advance decarbonization efforts over the coming decades.
Key Analysis Areas
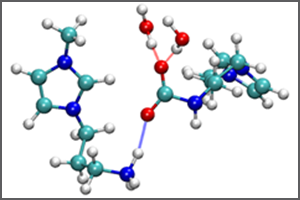
Solvent-based CO 2 capture involves chemical or physical absorption of CO 2 from a gas into a liquid carrier. R&D of advanced solvents (e.g., water-lean solvents, phase-change solvents, high-performance functionalized solvents) that have a lower regeneration energy requirement than existing amine systems, combined with high CO 2 absorption capacity and tolerance to impurities, is a key objective for lowering capture costs. System advancements include process intensification techniques, methods to mitigate aerosol formation and corrosion, and heat integration approaches.
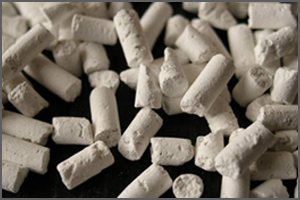
Sorbent-based CO 2 capture involves the chemical or physical adsorption of CO 2 from a gas using a solid sorbent. R&D objectives include low-cost durable sorbents that have high selectivity for CO 2 , high CO 2 adsorption capacity, resistance to oxidation, and can withstand multiple regeneration cycles with minimal attrition. System advancements include sorbent process intensification techniques, novel reactor designs, and enhanced process configurations, such as rotating beds for CO 2 adsorption and desorption.
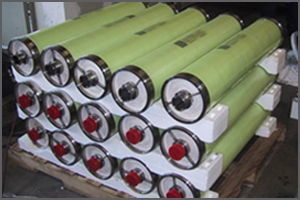
Membrane-based CO 2 capture uses permeable or semi-permeable materials that allow for the selective transport and separation of CO 2 from a gas. Membrane processes offer potential advantages when compared to other CO 2 separation technologies, including no hazardous chemical storage, handling, disposal, or emissions issues; simple passive operation; tolerance to high sulfur oxide [SOX] and nitrogen oxide [NOX] content; a reduced plant footprint; and efficient partial CO 2 capture. R&D objectives include development of low-cost, durable membranes (e.g., polymeric membranes, mixed matrix membranes, sub-ambient temperature membranes) that have improved permeability and selectivity for CO 2 , thermal and physical stability, and tolerance to gas contaminants. Process enhancements for membrane-based capture systems include low-pressure drop membrane modules.
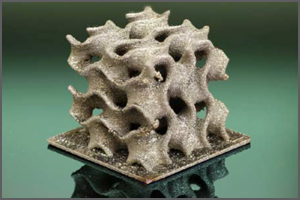
Novel Concepts
Novel concepts include alternative technologies and processes, such as cryogenic separation and electrochemical membranes, and additive manufacturing of novel system components and materials. R&D objectives include development of equipment, materials, and processes that enable intensified thermodynamic operations, improve process performance, and reduce equipment size, lowering capital and operating costs.
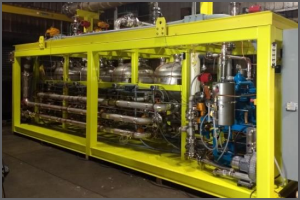
Hybrid systems efficiently combine two key technologies in a single system (e.g., sorbent-membrane system). Hybrid concepts can reduce the overall energy intake of the process by leveraging process synergies, resulting in a more cost-effective system.
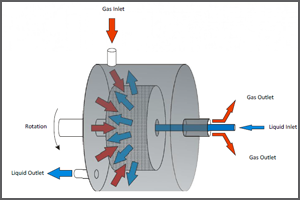
Enabling Technologies
Enabling technologies are concepts that could improve a whole class of materials, and although the research might be applied to one specific material, it is envisioned that substantial research findings could benefit multiple materials. R&D topics include solvent aerosol emissions mitigation, solvent viscosity reduction, solvent stability improvements, materials compatibility, corrosion resistance improvements, and degradation products reduction or separation.
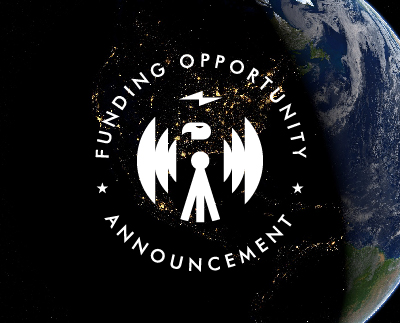
DOE To Invest $30 Million in Carbon Conversion and Highly Efficient CO 2 Capture Technologies FOA000-2614: CARBON MANAGEMENT: Funding will support the Nation’s commitment to using carbon emissions as a feedstock for value-added products and developing lower-cost, highly efficient technologies for point source CO 2 capture.
Request for Information: DE-FOA-0003263-Industrial Deployment and Demonstration Opportunities for Carbon Capture Technologies RFI seeking input to assist DOE in the planning of priorities and initiatives to catalyze the development, demonstration, and deployment of carbon capture, conversion, and storage technologies to decarbonize America’s industrial sectors.
DOE Office of Clean Energy Demonstrations (OCED) Selects Four Projects as Part of the Carbon Capture Large-Scale Pilot Projects Program DOE OCED announced up to $304 million in funding for four projects—in KY, MS, TX, and WY—as part of the Carbon Capture Large-Scale Pilot Projects Program, which supports projects that implement carbon capture technologies at the pilot scale across the power and industrial sectors.
DOE Office of Clean Energy Demonstrations (OCED) selects three projects as part of the Carbon Capture Demonstration Projects Program DOE OCED announced up to $890 million in funding for three projects — in CA, ND, and TX — as part of the Carbon Capture Demonstration Projects Program, which aims to advance integrated carbon capture, transport, and storage technologies and infrastructure that can be deployed at power plants and in hard-to-decarbonize heavy industries.
DOE/FECM issued NOI DE-FOA-0003232 for DE-FOA-0002614 titled Carbon Management (Round 5) Proposed Areas of Interest for Round 5 of DE-FOA-0002614 include R&D on technologies that utilize CO2 from point sources such as power/industrial flue gas and direct air capture to produce value-added products and the development of enabling technologies that support demonstration of carbon capture systems installed at electric generation or industrial facilities.
News: 2023 FECM / NETL Carbon Management Research Project Review Meeting Proceedings Posted The proceeding from the 2023 FECM / NETL Carbon Management Research Project Review Meeting held August 28 - September 1, 2023 are posted for the following programs: Point Source Carbon Capture, Carbon Dioxide Removal, Carbon Conversion, and Carbon Transport & Storage.
DOE/FECM Project Selections for Funding Opportunity Announcement 2614: Carbon Management (Round 3) $45.6 million in federal funding announced for nine projects that will advance carbon dioxide (CO 2 ) capture technologies and help build a foundation for a successful carbon transport and storage industry in the United States.

Explore the Site
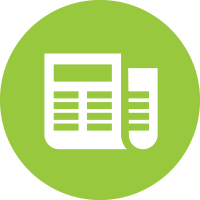
Project Information
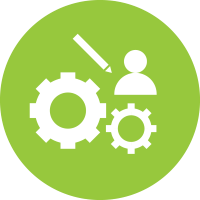
Systems Analysis
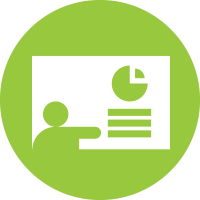
Infographics
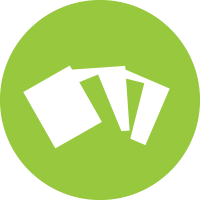
Publications
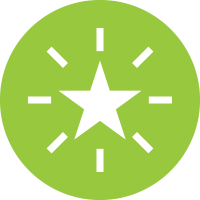
Accomplishments
- MyU : For Students, Faculty, and Staff
News Roundup Spring 2024
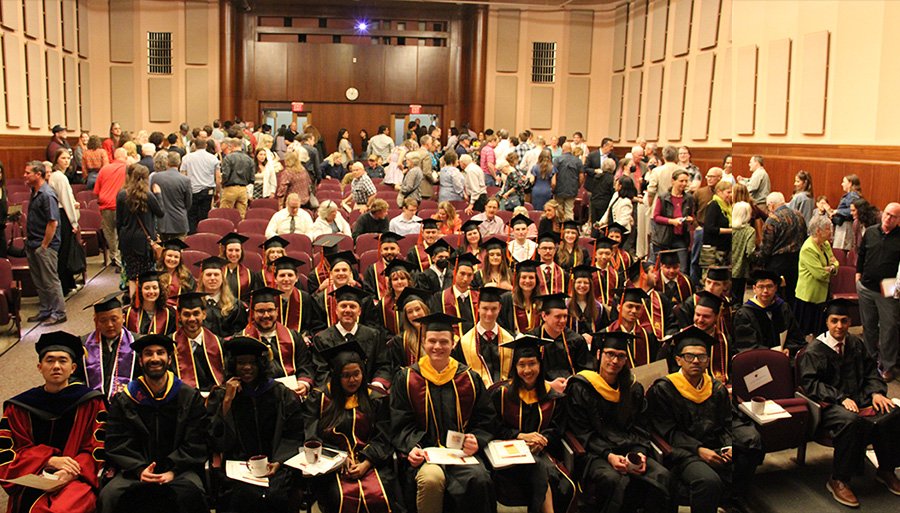
CEGE Spring Graduation Celebration and Order of the Engineer
Forty-seven graduates of the undergraduate and grad student programs (pictured above) in the Department of Civil, Environmental, and Geo- Engineering took part in the Order of the Engineer on graduation day. Distinguished Speakers at this departmental event included Katrina Kessler (MS EnvE 2021), Commissioner of the Minnesota Pollution Control Agency, and student Brian Balquist. Following this event, students participated in the college-wide Commencement Ceremony at 3M Arena at Mariucci.
UNIVERSITY & DEPARTMENT
The University of Minnesota’s Crookston, Duluth, and Rochester campuses have been awarded the Carnegie Elective Classification for Community Engagement, joining the Twin Cities (2006, 2015) and Morris campuses (2015), and making the U of M the country’s first and only university system at which every individual campus has received this selective designation. Only 368 from nearly 4,000 qualifying U.S. universities and colleges have been granted this designation.
CEGE contributed strongly to the College of Science and Engineering’s efforts toward sustainability research. CEGE researchers are bringing in over $35 million in funded research to study carbon mineralization, nature and urban areas, circularity of water resources, and global snowfall patterns. This news was highlighted in the Fall 2023 issue of Inventing Tomorrow (pages 10-11). https://issuu.com/inventingtomorrow/docs/fall_2023_inventing_tomorrow-web
CEGE’s new program for a one-year master’s degree in structural engineering is now accepting applicants for Fall 2024. We owe a big thanks to DAN MURPHY and LAURA AMUNDSON for their volunteer work to help curate the program with Professor JIA-LIANG LE and EBRAHIM SHEMSHADIAN, the program director. Potential students and companies interested in hosting a summer intern can contact Ebrahim Shemshadian ( [email protected] ).
BERNIE BULLERT , CEGE benefactor and MN Water Research Fund founder, was profiled on the website of the University of Minnesota Foundation (UMF). There you can read more about his mission to share clean water technologies with smaller communities in Minnesota. Many have joined Bullert in this mission. MWRF Recognizes their Generous 2024 Partners. Gold Partners: Bernie Bullert, Hawkins, Inc., Minnesota Department of Health, Minnesota Pollution Control Agency, and SL-serco. Silver Partners: ISG, Karl and Pam Streed, Kasco, Kelly Lange-Haider and Mark Haider, ME Simpson, Naeem Qureshi, Dr. Paul H. Boening, TKDA, and Waterous. Bronze Partners: Bruce R. Bullert; Brenda Lenz, Ph.D., APRN FNP-C, CNE; CDM Smith; Central States Water Environment Association (CSWEA MN); Heidi and Steve Hamilton; Jim “Bulldog” Sadler; Lisa and Del Cerney; Magney Construction; Sambatek; Shannon and John Wolkerstorfer; Stantec; and Tenon Systems.
After retiring from Baker-Tilly, NICK DRAGISICH (BCE 1977) has taken on a new role: City Council member in Lake Elmo, Minnesota. After earning his BCE from the University of Minnesota, Dragisich earned a master’s degree in business administration from the University of St. Thomas. Dragisich retired in May from his position as managing director at Baker Tilly, where he had previously served as firm director. Prior to that, he served as assistant city manager in Spokane, Washington, was the city administrator and city engineer in Virginia, Minnesota, and was mayor of Chisholm, Minnesota—all adding up to more than 40 years of experience in local government. Dragisich was selected by a unanimous vote. His current term expires in December 2024.
PAUL F. GNIRK (Ph.D. 1966) passed away January 29, 2024, at the age of 86. A memorial service was held Saturday, February 24, at the South Dakota School of Mines and Technology (SDSM&T), where he started and ended his teaching career, though he had many other positions, professional and voluntary. In 2018 Paul was inducted into the SDSM&T Hardrocker Hall of Fame, and in 2022, he was inducted into the South Dakota Hall of Fame, joining his mother Adeline S. Gnirk, who had been inducted in 1987 for her work authoring nine books on the history of south central South Dakota.
ROGER M. HILL (BCE 1957) passed away on January 13, 2024, at the age of 90. His daughter, Kelly Robinson, wrote to CEGE that Roger was “a dedicated Gopher fan until the end, and we enjoyed many football games together in recent years. Thank you for everything.”
KAUSER JAHAN (Ph.D. 1993, advised by Walter Maier), PE, is now a civil and environmental engineering professor and department head at Henry M. Rowan College of Engineering. Jahan was awarded a 3-year (2022- 2025), $500,000 grant from the U.S. Department of Environmental Protection Agency (USEPA). The grant supports her project, “WaterWorks: Developing the New Generation of Workforce for Water/Wastewater Utilities,” for the development of educational tools that will expose and prepare today’s students for careers in water and wastewater utilities.
SAURA JOST (BCE 2010, advised by Timothy LaPara) was elected to the St. Paul City Council for Ward 3. She is part of the historic group of women that make up the nation’s first all-female city council in a large city.
The 2024 ASCE Western Great Lakes Student Symposium combines several competitions for students involved in ASCE. CEGE sent a large contingent of competitors to Chicago. Each of the competition groups won awards: Ethics Paper 1st place Hans Lagerquist; Sustainable Solutions team 1st place overall in (qualifying them for the National competition in Utah in June); GeoWall 2nd place overall; Men’s Sprint for Concrete Canoe with rowers Sakthi Sundaram Saravanan and Owen McDonald 2nd place; Product Prototype for Concrete Canoe 2nd place; Steel Bridge (200 lb bridge weight) 2nd place in lightness; Scavenger Hunt 3rd place; and Aesthetics and Structural Efficiency for Steel Bridge 4th place.
Students competing on the Minnesota Environmental Engineers, Scientists, and Enthusiasts (MEESE) team earned second place in the Conference on the Environment undergraduate student design competition in November 2023. Erin Surdo is the MEESE Faculty Adviser. Pictured are NIKO DESHPANDE, ANNA RETTLER, and SYDNEY OLSON.
The CEGE CLASS OF 2023 raised money to help reduce the financial barrier for fellow students taking the Fundamentals of Engineering exam, a cost of $175 per test taker. As a result of this gift, they were able to make the exam more affordable for 15 current CEGE seniors. CEGE students who take the FE exam pass the first time at a rate well above national averages, demonstrating that CEGE does a great job of teaching engineering fundamentals. In 2023, 46 of 50 students passed the challenging exam on the first try.
This winter break, four CEGE students joined 10 other students from the College of Science and Engineering for the global seminar, Design for Life: Water in Tanzania. The students visited numerous sites in Tanzania, collected water source samples, designed rural water systems, and went on safari. Read the trip blog: http://globalblogs.cse.umn.edu/search/label/Tanzania%202024
Undergraduate Honor Student MALIK KHADAR (advised by Dr. Paul Capel) received honorable mention for the Computing Research Association (CRA) Outstanding Undergraduate Research Award for undergraduate students who show outstanding research potential in an area of computing research.
GRADUATE STUDENTS
AKASH BHAT (advised by William Arnold) presented his Ph.D. defense on Friday, October 27, 2023. Bhat’s thesis is “Photolysis of fluorochemicals: Tracking fluorine, use of UV-LEDs, and computational insights.” Bhat’s work investigating the degradation of fluorinated compounds will assist in the future design of fluorinated chemicals such that persistent and/or toxic byproducts are not formed in the environment.
ETHAN BOTMEN (advised by Bill Arnold) completed his Master of Science Final Exam February 28, 2024. His research topic was Degradation of Fluorinated Compounds by Nucleophilic Attack of Organo-fluorine Functional Groups.
XIATING CHEN , Ph.D. Candidate in Water Resources Engineering at the Saint Anthony Falls Laboratory is the recipient of the 2023 Nels Nelson Memorial Fellowship Award. Chen (advised by Xue Feng) is researching eco-hydrological functions of urban trees and other green infrastructure at both the local and watershed scale, through combined field observations and modeling approaches.
ALICE PRATES BISSO DAMBROZ has been a Visiting Student Researcher at the University of Minnesota since last August, on a Doctoral Dissertation Research Award from Fulbright. Her CEGE advisor is Dr. Paul Capel. Dambroz is a fourth year Ph.D. student in Soil Science at Universidade Federal de Santa Maria in Brazil, where she studies with her adviser Jean Minella. Her research focuses on the hydrological monitoring of a small agricultural watershed in Southern Brazil, which is located on a transition area between volcanic and sedimentary rocks. Its topography, shallow soils, and land use make it prone to runoff and erosion processes.
Yielding to people in crosswalks should be a very pedestrian topic. Yet graduate student researchers TIANYI LI, JOSHUA KLAVINS, TE XU, NIAZ MAHMUD ZAFRI (Dept.of Urban and Regional Planning at Bangladesh University of Engineering and Technology), and Professor Raphael Stern found that drivers often do not yield to pedestrians, but they are influenced by the markings around a crosswalk. Their work was picked up by the Minnesota Reformer.
TIANYI LI (Ph.D. student advised by Raphael Stern) also won the Dwight David Eisenhower Transportation (DDET) Fellowship for the third time! Li (center) and Stern (right) are pictured at the Federal Highway Administration with Latoya Jones, the program manager for the DDET Fellowship.
The Three Minute Thesis Contest and the Minnesota Nice trophy has become an annual tradition in CEGE. 2023’s winner was EHSANUR RAHMAN , a Ph.D. student advised by Boya Xiong.
GUANJU (WILLIAM) WEI , a Ph.D. student advised by Judy Yang, is the recipient of the 2023 Heinz G. Stefan Fellowship. He presented his research entitled Microfluidic Investigation of the Biofilm Growth under Dynamic Fluid Environments and received his award at the St. Anthony Falls Research Laboratory April 9. The results of Wei's research can be used in industrial, medical, and scientific fields to control biofilm growth.
BILL ARNOLD stars in an award-winning video about prairie potholes. The Prairie Potholes Project film was made with the University of Delaware and highlights Arnold’s NSF research. The official winners of the 2024 Environmental Communications Awards Competition Grand Prize are Jon Cox and Ben Hemmings who produced and directed the film. Graduate student Marcia Pacheco (CFANS/LAAS) and Bill Arnold are the on-screen stars.
Four faculty from CEGE join the Center for Transportation Studies Faculty and Research Scholars for FY24–25: SEONGJIN CHOI, KETSON ROBERTO MAXIMIANO DOS SANTOS, PEDRAM MORTAZAVI, and BENJAMIN WORSFOLD . CTS Scholars are drawn from diverse fields including engineering, planning, computer science, environmental studies, and public policy.
XUE FENG is coauthor on an article in Nature Reviews Earth and Environment . The authors evaluate global plant responses to changing rainfall regimes that are now characterized by fewer and larger rainfall events. A news release written at Univ. of Maryland can be found here: https://webhost.essic. umd.edu/april-showers-bring-mayflowers- but-with-drizzles-or-downpours/ A long-running series of U of M research projects aimed at improving stormwater quality are beginning to see practical application by stormwater specialists from the Twin Cities metro area and beyond. JOHN GULLIVER has been studying best practices for stormwater management for about 16 years. Lately, he has focused specifically on mitigating phosphorous contamination. His research was highlighted by the Center for Transportation Studies.
JIAQI LI, BILL ARNOLD, and RAYMOND HOZALSKI published a paper on N-nitrosodimethylamine (NDMA) precursors in Minnesota rivers. “Animal Feedlots and Domestic Wastewater Discharges are Likely Sources of N-Nitrosodimethylamine (NDMA) Precursors in Midwestern Watersheds,” Environmental Science and Technology (January 2024) doi: 10.1021/acs. est.3c09251
ALIREZA KHANI contributed to MnDOT research on Optimizing Charging Infrastructure for Electric Trucks. Electric options for medium- and heavy-duty electric trucks (e-trucks) are still largely in development. These trucks account for a substantial percentage of transportation greenhouse gas emissions. They have greater power needs and different charging needs than personal EVs. Proactively planning for e-truck charging stations will support MnDOT in helping to achieve the state’s greenhouse gas reduction goals. This research was featured in the webinar “Electrification of the Freight System in Minnesota,” hosted by the University of Minnesota’s Center for Transportation Studies. A recording of the event is now available online.
MICHAEL LEVIN has developed a unique course for CEGE students on Air Transportation Systems. It is the only class at UMN studying air transportation systems from an infrastructure design and management perspective. Spring 2024 saw the third offering of this course, which is offered for juniors, seniors, and graduate students.
Research Professor SOFIA (SONIA) MOGILEVSKAYA has been developing international connections. She visited the University of Seville, Spain, November 13–26, 2023, where she taught a short course titled “Fundamentals of Homogenization in Composites.” She also met with the graduate students to discuss collaborative research with Prof. Vladislav Mantic, from the Group of Continuum Mechanics and Structural Analysis at the University of Seville. Her visit was a part of planned activities within the DIAGONAL Consortium funded by the European Commission. CEGE UMN is a partner organization within DIAGONAL, represented by CEGE professors Mogilevskaya and Joseph Labuz. Mantic will visit CEGE summer 2024 to follow up on research developments and discuss plans for future collaboration and organization of short-term exchange visits for the graduate students from each institution.
DAVID NEWCOMB passed away in March. He was a professor in CEGE from 1989–99 in the area of pavement engineering. Newcomb led the research program on asphalt materials characterization. He was the technical director of Mn/ROAD pavement research facility, and he started an enduring collaboration with MnDOT that continues today. In 2000, he moved from Minnesota to become vice-president for Research and Technology at the National Asphalt Pavement Association. Later he moved to his native Texas, where he was appointed to the division head of Materials and Pavement at the Texas A&M Transportation Institute, a position from which he recently retired. He will be greatly missed.
PAIGE NOVAK won Minnesota ASCE’s 2023 Distinguished Engineer of the Year Award for her contributions to society through her engineering achievements and professional experiences.
The National Science Foundation (NSF) announced ten inaugural (NSF) Regional Innovation Engines awards, with a potential $1.6 billion investment nationally over the next decade. Great Lakes ReNEW is led by the Chicago-based water innovation hub, Current, and includes a team from the University of Minnesota, including PAIGE NOVAK. Current will receive $15 mil for the first two years, and up to $160 million over ten years to develop and grow a water-focused innovation engine in the Great Lakes region. The project’s ambitious plan is to create a decarbonized circular “blue economy” to leverage the region’s extraordinary water resources to transform the upper Midwest—Illinois, Indiana, Michigan, Minnesota, Ohio, and Wisconsin. Brewing one pint of beer generates seven pints of wastewater, on average. So what can you do with that wastewater? PAIGE NOVAK and her team are exploring the possibilities of capturing pollutants in wastewater and using bacteria to transform them into energy.
BOYA XIONG has been selected as a recipient of the 2024 40 Under 40 Recognition Program by the American Academy of Environmental Engineers and Scientists. The award was presented at the 2024 AAEES Awards Ceremony, April 11, 2024, at the historic Howard University in Washington, D.C.
JUDY Q. YANG received a McKnight Land-Grant Professorship Award. This two-year award recognizes promising assistant professors and is intended to advance the careers of individuals who have the potential to make significant contributions to their departments and their scholarly fields.
Professor Emeritus CHARLES FAIRHURST , his son CHARLES EDWARD FAIRHURST , and his daughter MARGARET FAIRHURST DURENBERGER were on campus recently to present Department Head Paige Novak with a check for $25,000 for the Charles Fairhurst Fellowship in Earth Resources Engineering in support of graduate students studying geomechanics. The life of Charles Fairhurst through a discussion with his children is featured on the Engineering and Technology History Wiki at https://ethw.org/Oral-History:Charles_Fairhurst#00:00:14_INTRODUCTION
Related news releases
- Matthew J. Huber Student Award
- Catherine French, NAE
- Climate Change for Engineers
- Focused on the Road Ahead
- Randal Barnes receives Horace T Morace Award
- Future undergraduate students
- Future transfer students
- Future graduate students
- Future international students
- Diversity and Inclusion Opportunities
- Learn abroad
- Living Learning Communities
- Mentor programs
- Programs for women
- Student groups
- Visit, Apply & Next Steps
- Information for current students
- Departments and majors overview
- Departments
- Undergraduate majors
- Graduate programs
- Integrated Degree Programs
- Additional degree-granting programs
- Online learning
- Academic Advising overview
- Academic Advising FAQ
- Academic Advising Blog
- Appointments and drop-ins
- Academic support
- Commencement
- Four-year plans
- Honors advising
- Policies, procedures, and forms
- Career Services overview
- Resumes and cover letters
- Jobs and internships
- Interviews and job offers
- CSE Career Fair
- Major and career exploration
- Graduate school
- Collegiate Life overview
- Scholarships
- Diversity & Inclusivity Alliance
- Anderson Student Innovation Labs
- Information for alumni
- Get engaged with CSE
- Upcoming events
- CSE Alumni Society Board
- Alumni volunteer interest form
- Golden Medallion Society Reunion
- 50-Year Reunion
- Alumni honors and awards
- Outstanding Achievement
- Alumni Service
- Distinguished Leadership
- Honorary Doctorate Degrees
- Nobel Laureates
- Alumni resources
- Alumni career resources
- Alumni news outlets
- CSE branded clothing
- International alumni resources
- Inventing Tomorrow magazine
- Update your info
- CSE giving overview
- Why give to CSE?
- College priorities
- Give online now
- External relations
- Giving priorities
- CSE Dean's Club
- Donor stories
- Impact of giving
- Ways to give to CSE
- Matching gifts
- CSE directories
- Invest in your company and the future
- Recruit our students
- Connect with researchers
- K-12 initiatives
- Diversity initiatives
- Research news
- Give to CSE
- CSE priorities
- Corporate relations
- Information for faculty and staff
- Administrative offices overview
- Office of the Dean
- Academic affairs
- Finance and Operations
- Communications
- Human resources
- Undergraduate programs and student services
- CSE Committees
- CSE policies overview
- Academic policies
- Faculty hiring and tenure policies
- Finance policies and information
- Graduate education policies
- Human resources policies
- Research policies
- Research overview
- Research centers and facilities
- Research proposal submission process
- Research safety
- Award-winning CSE faculty
- National academies
- University awards
- Honorary professorships
- Collegiate awards
- Other CSE honors and awards
- Staff awards
- Performance Management Process
- Work. With Flexibility in CSE
- K-12 outreach overview
- Summer camps
- Outreach events
- Enrichment programs
- Field trips and tours
- CSE K-12 Virtual Classroom Resources
- Educator development
- Sponsor an event
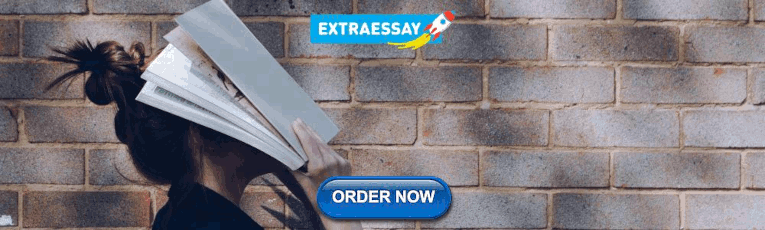
COMMENTS
How best to meet these challenges requires research in all aspects of water management. Since 1965, the journal Water Resources Research has played an important role in reporting and disseminating current research related to managing the quantity and quality and cost of this resource. ... This paper identifies the issues facing water managers ...
In this paper, various components of IoT based water management systems were presented along with the in-depth survey of all existing smart water management systems. Various measurement parameters such as water level, pH level, turbidity, salinity, etc. were also identified and a comparison of all existing systems based on these parameters has ...
Smart Water Management System. Julius Femi Godslove. Compunet Limited Rwang Pam St, 930105, Jos, Nigeria, [email protected]. Abstract. Water is one of the fundamental resources that aid ...
Issues such as climate change, water scarcity, population growth, and distribution losses have stimulated the use of new technologies to manage water resources. This is how the concept of smart water management emerged as a subcategory of the concept of smart cities. This article aimed first to identify the applications of smart water-management systems described in academic articles either as ...
The Internet of Things (IoT) sustainable solutions are the next generation of methods for managing and monitoring valuable natural resources such as water. Research has focused on smart IoT-based water management and monitoring system designs for various types of applications, including agricultural, industrial, residential, and crude oil exploration sectors. To this end, unlike other surveys ...
on smart water technologies implemented in urban contexts, emphasizing supply and distribution. networks. The review analysis outcomes were classified according to three main pillars identified ...
Smart W ater T echnology for Ef ficient W ater Resource. Management: A Review. Aditya Dinesh Gupta 1, Prerna Pandey 2, Andrés Feijóo 3, Zaher Mundher Yaseen 4. and Neeraj Dhanraj Bokde 5,∗. 1 ...
Development of research on water quality management integrated with recent ad-vances in IoT technology continuously is the pinnacle for this research as it aims to con-duct a systematic literature review to examine the latest research trends on water quality management. It provides valuable insights into technological environments and support
Water quality monitoring plays a significant part in the transition towards intelligent and smart agriculture and provides an easy transition to automated monitoring of crucial components of human daily needs as new technologies are continuously developed and adopted in agricultural and human daily life (water). For the monitoring and management of water quality, this effort, however, requires ...
Water management is one of the crucial topics discussed in most of the international forums. Water harvesting and recycling are the major requirements to meet the global upcoming demand of the water crisis, which is prevalent. To achieve this, we need more emphasis on water management techniques that are applied across various categories of the applications. Keeping in mind the population ...
In water environment research, models and conclusions derived from machine learning have been applied to the construction, monitoring, simulation, evaluation, and optimization of various water treatment and management systems. ... As the conditions in real water treatment and management systems can be extremely complex, the current algorithms ...
The research paper presents a groundbreaking contribution by introducing the "Advanced Irrigation System" to tackle the pressing issue of optimizing water and power usage in agriculture. This innovative solution encompasses several key features, including...
This paper presents a plot holding level plan for smart water management. Applicable in upcoming smart cities [ 4 ], this IoT- and DA-based system uses user consumption behavior to optimize the water distribution. Using smart sensors, the proposed system also presents a plan to harvest rainwater at the plot level.
The system has greatly improved all the sanitation system management but will face significant challenges in the future, with probable reduction of the flow of the Seine due to climate change. Continuous improvements, enabled by technological advance, facilitating even smarter water management, are foreseeable and necessary.
Water management in post-2020 world. The year 2020 will go down in history as one of the most challenging ones for humankind from all points of view due to COVID-19 and the accelerating impacts of climate change. Both have caused, and will continue to cause, both directly and indirectly, severe disruptions of global economic activities, overall ...
The latest progress in information and communication technology (ICT) and the Internet of Things (IoT) have opened up new opportunities for real-time monitoring and controlling of cities' structures, infrastructures, and services. In this context, smart water management technology provides the data and tools to help users more effectively manage water usage. Data collected with smart water ...
Research Importance: The paper tackles and highlights the sustainable methods, techniques, ... An integrated water management system can also offer benefits in terms of energy efficiency. For example, green roof systems increase the thermal efficiency of the roof. Many water efficiency technologies provide not only water saving, but also energy ...
Vijayan et al. have analyzed basic services in smart buildings (security control, energy management, control and monitoring of HVAC systems, water management, lighting systems, fire detection, health systems, and elderly care) to provide a guideline for future research. Other reviews focused on specific methods for leakage detection and ...
This paper presents an IOT device which help to manage and plan the usage of water. This system can be easily installed in residential societies. Sensors placed in the tank which continuously informs the water level at the current time. This information will be updated on the cloud and using an android application, user can visualize the water level on a Smartphone anywhere that is connected ...
Feature papers represent the most advanced research with significant potential for high impact in the field. A Feature Paper should be a substantial original Article that involves several techniques or approaches, provides an outlook for future research directions and describes possible research applications. ... "Applications of Smart Water ...
Solvents. Solvent-based CO 2 capture involves chemical or physical absorption of CO 2 from a gas into a liquid carrier. R&D of advanced solvents (e.g., water-lean solvents, phase-change solvents, high-performance functionalized solvents) that have a lower regeneration energy requirement than existing amine systems, combined with high CO 2 absorption capacity and tolerance to impurities, is a ...
Integrated Urban Water Management (IUWM) is a concept that addresses water management (water supply, storm water, wastewater, etc.) in urban areas with a comprehensive approach (Bahri, 2012).
CEGE Spring Graduation Celebration and Order of the EngineerForty-seven graduates of the undergraduate and grad student programs (pictured above) in the Department of Civil, Environmental, and Geo- Engineering took part in the Order of the Engineer on graduation day. Distinguished Speakers at this departmental event included Katrina Kessler (MS EnvE 2021), Commissioner of the Minnesota ...
Water is a precious resource that can be intelligently managed. Effective water usage demands computerized home water supply management in a culture where water tanks, motors, and pumps are ubiquitous. Water management is crucial for the government and the citizens in countries like Saudi Arabia. The issue is providing a constant, high-quality, low-cost water supply. This study introduces a ...
Access the portal of NASS, the official source of agricultural data and statistics in the US, and explore various reports and products.
The authors annually evaluated the reduction in the use of water (2.5 10 5 tons), nitrogen (5.6 NH 3 -N tons), and carbon emissions (134 tons) through the reuse of treated sewage. Advances in the management of solid waste and wastewater treatment include landfilling, the composting of organic matter, reductions in greenhouse gas emissions and ...