Lock-and-key model
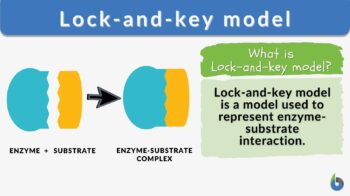
strong>Lock-and-key model n., [lɑk ænd ki ˈmɑdl̩] Definition: a model for enzyme-substrate interaction
Table of Contents
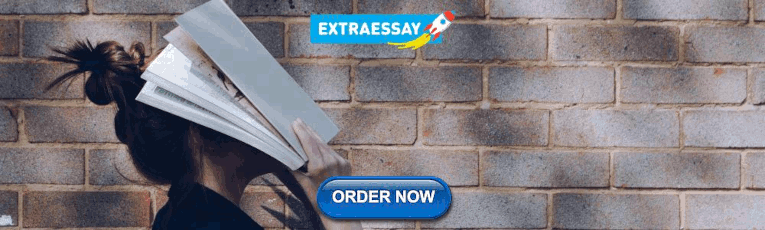
Lock-and-key model Definition
Lock-and-key model is a model for enzyme-substrate interaction suggesting that the enzyme and the substrate possess specific complementary geometric shapes that fit exactly into one another. In this model, enzymes are depicted as highly specific. They must bind to specific substrates before they catalyze chemical reactions . The term is a pivotal concept in enzymology to elucidate the intricate interaction between enzymes and substrates at the molecular level. In the lock-and-key model, the enzyme-substrate interaction suggests that the enzyme and the substrate possess specific complementary geometric shapes that fit exactly into one another. Like a key into a lock , only the correct size and shape of the substrate ( the key ) would fit into the active site ( the keyhole ) of the enzyme ( the lock ).
Compare: Induced fit model See also: enzyme , active site , substrate
Lock-and-key vs. Induced Fit Model
At present, two models attempt to explain enzyme-substrate specificity; one of which is the lock-and-key model , and the other is the Induced fit model . The lock and key model theory was first postulated by Emil Fischer in 1894. The lock-and-key enzyme action proposes the high specificity of enzymes. However, it does not explain the stabilization of the transition state that the enzymes achieve. The induced fit model (proposed by Daniel Koshland in 1958) suggests that the active site continues to change until the substrate is completely bound to the active site of the enzyme, at which point the final shape and charge are determined. Unlike the lock-and-key model, the induced fit model shows that enzymes are rather flexible structures. Nevertheless, Fischer’s Lock and Key theory laid an important foundation for subsequent research, such as during the refinement of the enzyme-substrate complex mechanism, as ascribed in the induced fit model. The lock-and-key hypothesis has opened ideas where enzyme action is not merely catalytic but incorporates a rather complex process in how they interact with the correct substrates with precision.
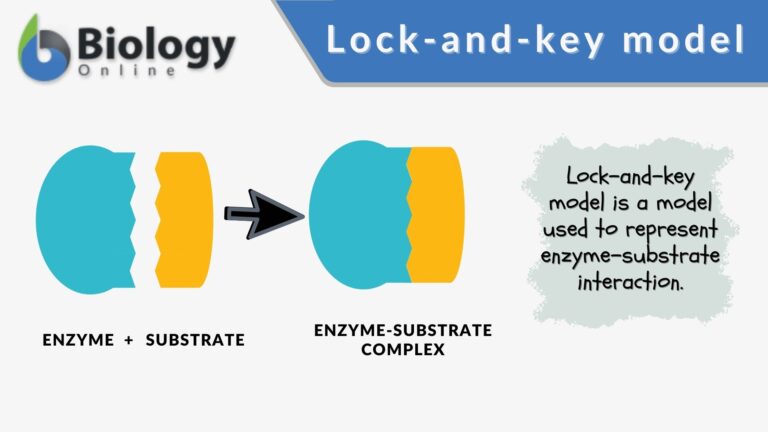
Key Components
Components of the lock and key model:
- Enzyme : the enzyme structure is a three-dimensional protein configuration, with an active site from where the substrate binds.
- Substrate : often an organic molecule, a substrate possesses a structural feature that complements the geometry of the enzyme’s active site.
In the lock and key model, both the enzymes and the substrates facilitate the formation of a complex that lowers the activation energy needed for a chemical transformation to occur. Such reduction in the activation energy allows the chemical reaction to proceed at a relatively faster rate, making enzymes crucial in various biological and molecular processes.
Lock-and-key Model Examples
Some of the common examples that are often discussed in the context of the Lock and Key Model are as follows:
- Enzyme lactate dehydrogenase with a specific active site for its substrates, pyruvate and lactate. The complex facilitates the interconversion of pyruvate and lactate during anaerobic respiration
- Enzyme carbonic anhydrase with a specific active site for the substrates carbon dioxide and water. The complex facilitates the hydration of carbon dioxide, forming bicarbonate
- Enzyme lysozyme binding with a bacterial cell wall peptidoglycan, which is a vital immune function
Choose the best answer.
Send Your Results (Optional)
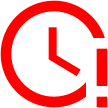
- Aryal, S. and Karki, P. (2023). “Lock and Key Model- Mode of Action of Enzymes”. Microbenotes.com. https://microbenotes.com/lock-and-key-model-mode-of-action-of-enzymes/
- Farhana, A., & Lappin, S. L. (2023, May). Biochemistry, Lactate Dehydrogenase . Nih.gov; StatPearls Publishing. https://www.ncbi.nlm.nih.gov/books/NBK557536/
©BiologyOnline.com. Content provided and moderated by Biology Online Editors.
Last updated on January 11th, 2024
You will also like...
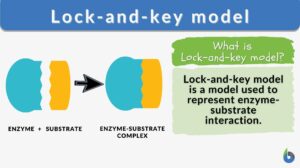
Primitive Animals
Life, as we know it today, is presumed to have started in the sea and many of them were likely eukaryotic animal-like or..
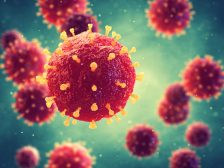
Biological Viruses
Viruses possess both living and non-living characteristics. This unique feature distinguishes them from other organisms...
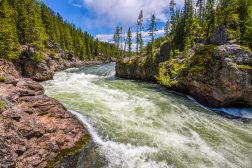
Running Water Freshwater Communities
This tutorial introduces flowing water communities, which bring new and dithering factors into the equation for possible..
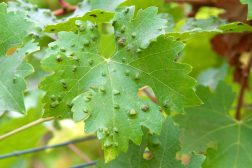
Plant Cell Defense
Plants protect themselves by releasing hydrogen peroxide to fight against fungal invasion. Another way is by secreting c..
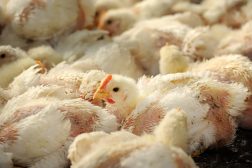
Selective Breeding
Gregor Mendel's studies into Monohybrid and Dihybrid crossing and Charles Darwin's study of evolution and natural select..
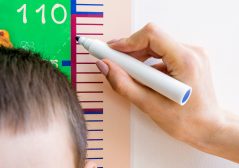
Physical Development in Humans
This tutorial elaborates on the physical development of humans, particularly from puberty to adulthood. Read this tutori..
Related Articles...
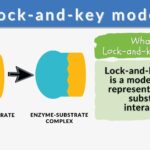
No related articles found
Molecular Recognition: Lock-and-Key, Induced Fit, and Conformational Selection
- Reference work entry
- pp 1584–1588
- Cite this reference work entry
- Todd Holyoak 2
1499 Accesses
6 Citations
Fluctuation fit ; Population selection ; Population shift ; Preexisting equilibrium ; Selected fit
In the most general sense, molecular recognition corresponds to the mechanism by which two or more molecules come together to form a specific complex. These types of specific molecular interactions span biology and include processes as diverse as enzyme catalysis, antibody–antigen recognition, protein synthesis, and transcriptional regulation to name but a few. As a consequence of the universal importance of molecular recognition in biological function, understanding how molecules specifically recognize and interact with one another is of fundamental importance.
Introduction
Early studies on the kinetics and specificity of enzyme-catalyzed reactions led to the conclusion that substrates combine with the enzyme at a specific active site location on the enzyme surface. This understanding generated new questions as to how enzymes could carry out chemical transformations...
This is a preview of subscription content, log in via an institution to check access.
Access this chapter
- Available as PDF
- Read on any device
- Instant download
- Own it forever
- Available as EPUB and PDF
- Durable hardcover edition
- Dispatched in 3 to 5 business days
- Free shipping worldwide - see info
Tax calculation will be finalised at checkout
Purchases are for personal use only
Institutional subscriptions
Beach H, Cole R, Gill ML, Loria JP. Conservation of mus-ms enzyme motions in the apo- and substrate-mimicked state. J Am Chem Soc. 2005;127(25):9167–76.
CAS PubMed Google Scholar
Csermely P, Palotai R, Nussinov R. Induced fit, conformational selection and independent dynamic segments: an extended view of binding events. Trends Biochem Sci. 2010;35(10):539–46.
CAS PubMed Central PubMed Google Scholar
Dixon M, Webb EC. Enzymes. New York: Academic; 1979.
Google Scholar
Fischer E. The influence of configuration on enzyme activity. Dtsch Chem Ges. 1894;27:2984–93 (Translated from German).
Gerstein M, Lesk AM, Chothia C. Structural mechanisms for domain movements in proteins. Biochemistry. 1994;33(22):6739–49.
Jencks WP. Binding energy, specificity, and enzymic catalysis: the circe effect. Adv Enzymol Relat Areas Mol Biol. 1975;43:219–410.
Koshland DE. Application of a theory of enzyme specificity to protein synthesis. Proc Natl Acad Sci USA. 1958;44(2):98–104.
Koshland Jr DE. Crazy, but correct. Nature. 2004;432(7016):447.
Laidler KH. The influence of pressure on the rates of biological reactions. Arch Biochem. 1951;30(2):226–36.
Monod J, Wyman J, Changeux JP. On the nature of allosteric transitions: a plausible model. J Mol Biol. 1965;12:88–118.
Sullivan SM, Holyoak T. Enzymes with lid-gated active sites must operate by an induced fit mechanism instead of conformational selection. Proc Natl Acad Sci USA. 2008;105(37):13829–34.
Tsai CJ, Kumar S, Ma B, Nussinov R. Folding funnels, binding funnels, and protein function. Protein Sci. 1999a;8(6):1181–90.
Tsai CJ, Ma B, Nussinov R. Folding and binding cascades: shifts in energy landscapes. Proc Natl Acad Sci USA. 1999b;96(18):9970–2.
Voet D, Voet JG. Biochemistry. Hoboken: Wiley; 2004.
Download references
Author information
Authors and affiliations.
Department of Biochemistry and Molecular Biology, The University of Kansas Medical Center, 3901 Rainbow Boulevard, Kansas City, KS, 66160-7421, USA
Todd Holyoak
You can also search for this author in PubMed Google Scholar
Corresponding author
Correspondence to Todd Holyoak .
Editor information
Editors and affiliations.
Department of Biochemistry, University of Leicester, Leicester, UK
Gordon C. K. Roberts
Rights and permissions
Reprints and permissions
Copyright information
© 2013 European Biophysical Societies' Association (EBSA)
About this entry
Cite this entry.
Holyoak, T. (2013). Molecular Recognition: Lock-and-Key, Induced Fit, and Conformational Selection. In: Roberts, G.C.K. (eds) Encyclopedia of Biophysics. Springer, Berlin, Heidelberg. https://doi.org/10.1007/978-3-642-16712-6_468
Download citation
DOI : https://doi.org/10.1007/978-3-642-16712-6_468
Publisher Name : Springer, Berlin, Heidelberg
Print ISBN : 978-3-642-16711-9
Online ISBN : 978-3-642-16712-6
eBook Packages : Biomedical and Life Sciences Reference Module Biomedical and Life Sciences
Share this entry
Anyone you share the following link with will be able to read this content:
Sorry, a shareable link is not currently available for this article.
Provided by the Springer Nature SharedIt content-sharing initiative
- Publish with us
Policies and ethics
- Find a journal
- Track your research
Structural Biochemistry/Protein function/Lock and Key
In the Lock and Key Model, first presented by Emil Fisher, the lock represents an enzyme and the key represents a substrate. It is assumed that both the enzyme and substrate have fixed conformations that lead to an easy fit. Because the enzyme and the substrate are at a close distance with weak attraction, the substrate must need a matching shape and fit to join together. At the active sites, the enzyme has a specific geometric shape and orientation that a complementary substrate fits into perfectly. The theory behind the Lock and Key model involves the complementarity between the shapes of the enzyme and the substrate. Their complementary shapes make them fit perfectly into each other like a lock and a key. According to this theory, the enzyme and substrate shape do not influence each other because they are already in a predetermined perfectly complementary shape. As a result, the substrate will be stabilized. This theory was replaced by the induced fit model which takes into account the flexibility of enzymes and the influence the substrate has on the shape of the enzyme in order to form a good fit.

The active site is the binding site for catalytic and inhibition reaction of the enzyme and the substrate; structure of active site and its chemical characteristic are of specificity for binding of substrate and enzyme. Three models of enzyme-substrate binding are the lock-and-key model, the induced fit model, and the transition-state model. The lock-and-key model assumes that active site of enzyme is good fit for substrate that does not require change of structure of enzyme after enzyme binds substrate.
- Book:Structural Biochemistry
Navigation menu
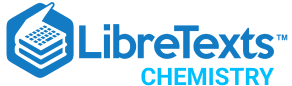
- school Campus Bookshelves
- menu_book Bookshelves
- perm_media Learning Objects
- login Login
- how_to_reg Request Instructor Account
- hub Instructor Commons
Margin Size
- Download Page (PDF)
- Download Full Book (PDF)
- Periodic Table
- Physics Constants
- Scientific Calculator
- Reference & Cite
- Tools expand_more
- Readability
selected template will load here
This action is not available.
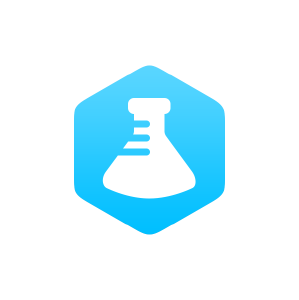
4.7: Enzyme Action
- Last updated
- Save as PDF
- Page ID 178782
\( \newcommand{\vecs}[1]{\overset { \scriptstyle \rightharpoonup} {\mathbf{#1}} } \)
\( \newcommand{\vecd}[1]{\overset{-\!-\!\rightharpoonup}{\vphantom{a}\smash {#1}}} \)
\( \newcommand{\id}{\mathrm{id}}\) \( \newcommand{\Span}{\mathrm{span}}\)
( \newcommand{\kernel}{\mathrm{null}\,}\) \( \newcommand{\range}{\mathrm{range}\,}\)
\( \newcommand{\RealPart}{\mathrm{Re}}\) \( \newcommand{\ImaginaryPart}{\mathrm{Im}}\)
\( \newcommand{\Argument}{\mathrm{Arg}}\) \( \newcommand{\norm}[1]{\| #1 \|}\)
\( \newcommand{\inner}[2]{\langle #1, #2 \rangle}\)
\( \newcommand{\Span}{\mathrm{span}}\)
\( \newcommand{\id}{\mathrm{id}}\)
\( \newcommand{\kernel}{\mathrm{null}\,}\)
\( \newcommand{\range}{\mathrm{range}\,}\)
\( \newcommand{\RealPart}{\mathrm{Re}}\)
\( \newcommand{\ImaginaryPart}{\mathrm{Im}}\)
\( \newcommand{\Argument}{\mathrm{Arg}}\)
\( \newcommand{\norm}[1]{\| #1 \|}\)
\( \newcommand{\Span}{\mathrm{span}}\) \( \newcommand{\AA}{\unicode[.8,0]{x212B}}\)
\( \newcommand{\vectorA}[1]{\vec{#1}} % arrow\)
\( \newcommand{\vectorAt}[1]{\vec{\text{#1}}} % arrow\)
\( \newcommand{\vectorB}[1]{\overset { \scriptstyle \rightharpoonup} {\mathbf{#1}} } \)
\( \newcommand{\vectorC}[1]{\textbf{#1}} \)
\( \newcommand{\vectorD}[1]{\overrightarrow{#1}} \)
\( \newcommand{\vectorDt}[1]{\overrightarrow{\text{#1}}} \)
\( \newcommand{\vectE}[1]{\overset{-\!-\!\rightharpoonup}{\vphantom{a}\smash{\mathbf {#1}}}} \)
Learning Objectives
- To describe the interaction between an enzyme and its substrate.
Enzyme-catalyzed reactions occur in at least two steps. In the first step, an enzyme molecule (E) and the substrate molecule or molecules (S) collide and react to form an intermediate compound called the enzyme-substrate (E–S) complex . (This step is reversible because the complex can break apart into the original substrate or substrates and the free enzyme.) Once the E–S complex forms, the enzyme is able to catalyze the formation of product (P), which is then released from the enzyme surface:
\[S + E \rightarrow E–S \tag{\(\PageIndex{1}\)}\]
\[E–S \rightarrow P + E \tag{\(\PageIndex{2}\)}\]
Hydrogen bonding and other electrostatic interactions hold the enzyme and substrate together in the complex. The structural features or functional groups on the enzyme that participate in these interactions are located in a cleft or pocket on the enzyme surface. This pocket, where the enzyme combines with the substrate and transforms the substrate to product is called the active site of the enzyme (Figure \(\PageIndex{1}\)).
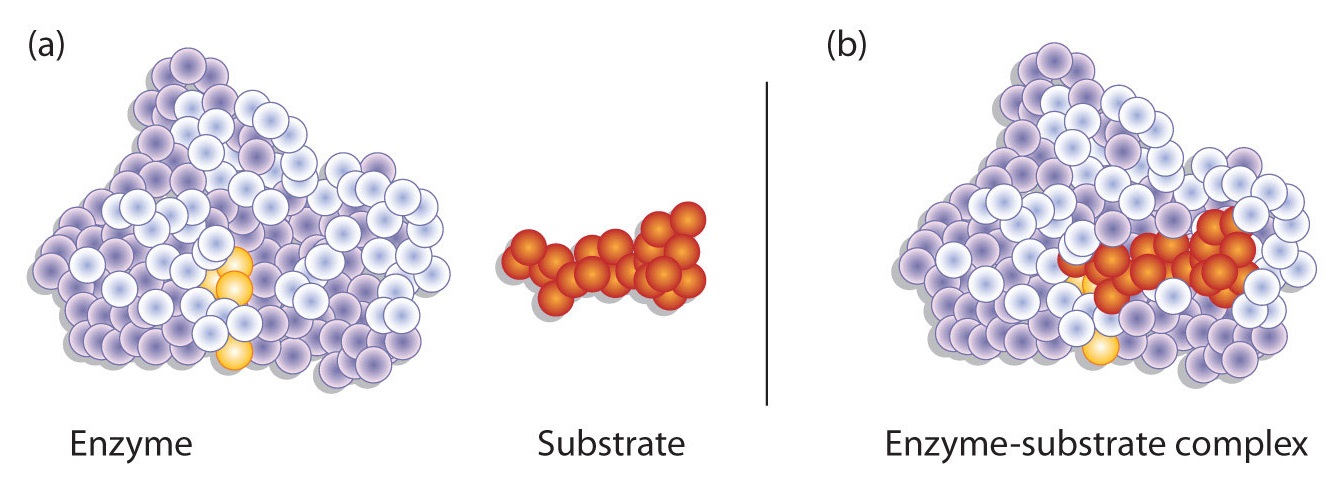
The active site of an enzyme possesses a unique conformation (including correctly positioned bonding groups) that is complementary to the structure of the substrate, so that the enzyme and substrate molecules fit together in much the same manner as a key fits into a tumbler lock. In fact, an early model describing the formation of the enzyme-substrate complex was called the lock-and-key model (Figure \(\PageIndex{2}\)). This model portrayed the enzyme as conformationally rigid and able to bond only to substrates that exactly fit the active site.

Working out the precise three-dimensional structures of numerous enzymes has enabled chemists to refine the original lock-and-key model of enzyme actions. They discovered that the binding of a substrate often leads to a large conformational change in the enzyme, as well as to changes in the structure of the substrate or substrates. The current theory, known as the induced-fit model , says that enzymes can undergo a change in conformation when they bind substrate molecules, and the active site has a shape complementary to that of the substrate only after the substrate is bound, as shown for hexokinase in Figure \(\PageIndex{3}\). After catalysis, the enzyme resumes its original structure.
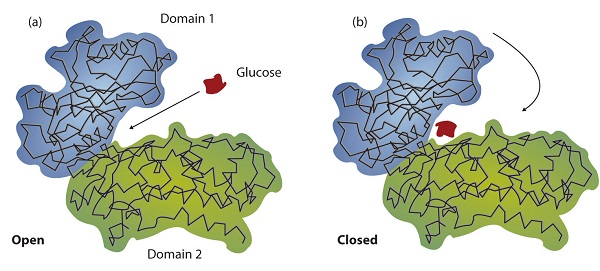
The structural changes that occur when an enzyme and a substrate join together bring specific parts of a substrate into alignment with specific parts of the enzyme’s active site. Amino acid side chains in or near the binding site can then act as acid or base catalysts, provide binding sites for the transfer of functional groups from one substrate to another or aid in the rearrangement of a substrate. The participating amino acids, which are usually widely separated in the primary sequence of the protein, are brought close together in the active site as a result of the folding and bending of the polypeptide chain or chains when the protein acquires its tertiary and quaternary structure. Binding to enzymes brings reactants close to each other and aligns them properly, which has the same effect as increasing the concentration of the reacting compounds.
Example \(\PageIndex{1}\)
- What type of interaction would occur between an OH group present on a substrate molecule and a functional group in the active site of an enzyme?
- Suggest an amino acid whose side chain might be in the active site of an enzyme and form the type of interaction you just identified.
- An OH group would most likely engage in hydrogen bonding with an appropriate functional group present in the active site of an enzyme.
- Several amino acid side chains would be able to engage in hydrogen bonding with an OH group. One example would be asparagine, which has an amide functional group.
Exercise \(\PageIndex{1}\)
- What type of interaction would occur between an COO − group present on a substrate molecule and a functional group in the active site of an enzyme?
One characteristic that distinguishes an enzyme from all other types of catalysts is its substrate specificity . An inorganic acid such as sulfuric acid can be used to increase the reaction rates of many different reactions, such as the hydrolysis of disaccharides, polysaccharides, lipids, and proteins, with complete impartiality. In contrast, enzymes are much more specific. Some enzymes act on a single substrate, while other enzymes act on any of a group of related molecules containing a similar functional group or chemical bond. Some enzymes even distinguish between D- and L-stereoisomers, binding one stereoisomer but not the other. Urease, for example, is an enzyme that catalyzes the hydrolysis of a single substrate—urea—but not the closely related compounds methyl urea, thiourea, or biuret. The enzyme carboxypeptidase, on the other hand, is far less specific. It catalyzes the removal of nearly any amino acid from the carboxyl end of any peptide or protein.
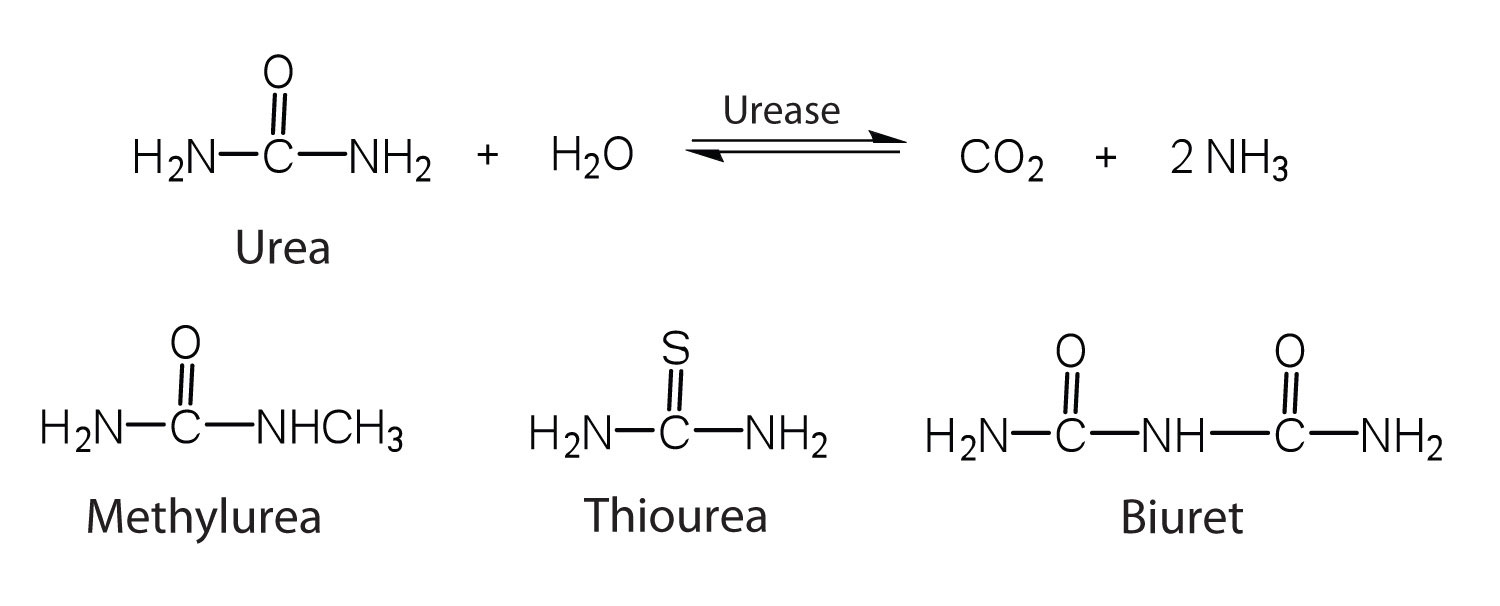
Enzyme specificity results from the uniqueness of the active site in each different enzyme because of the identity, charge, and spatial orientation of the functional groups located there. It regulates cell chemistry so that the proper reactions occur in the proper place at the proper time. Clearly, it is crucial to the proper functioning of the living cell.
A substrate binds to a specific region on an enzyme known as the active site, where the substrate can be converted to product. The substrate binds to the enzyme primarily through hydrogen bonding and other electrostatic interactions. The induced-fit model says that an enzyme can undergo a conformational change when binding a substrate. Enzymes exhibit varying degrees of substrate specificity.
Concept Review Exercises
- Distinguish between the lock-and-key model and induced-fit model of enzyme action.
- Which enzyme has greater specificity—urease or carboxypeptidase? Explain.
- The lock-and-key model portrays an enzyme as conformationally rigid and able to bond only to substrates that exactly fit the active site. The induced fit model portrays the enzyme structure as more flexible and is complementary to the substrate only after the substrate is bound.
- Urease has the greater specificity because it can bind only to a single substrate. Carboxypeptidase, on the other hand, can catalyze the removal of nearly any amino acid from the carboxyl end of a peptide or protein.
What type of interaction would occur between each group present on a substrate molecule and a functional group of the active site in an enzyme?
- CH(CH 3 ) 2
- COO −
For each functional group in Exercise 1, suggest an amino acid whose side chain might be in the active site of an enzyme and form the type of interaction you identified.
For each functional group in Exercise 2, suggest an amino acid whose side chain might be in the active site of an enzyme and form the type of interaction you identified.
- hydrogen bonding
- ionic bonding
- dispersion forces
- The amino acid has a polar side chain capable of engaging in hydrogen bonding; serine (answers will vary).
- The amino acid has a negatively charged side chain; aspartic acid (answers will vary).
- The amino acid has a polar side chain capable of engaging in hydrogen bonding; asparagine (answers will vary).
- The amino acid has a nonpolar side chain; isoleucine (answers will vary).
Lock and Key Model Enzyme Explained!
by Taha Cheema
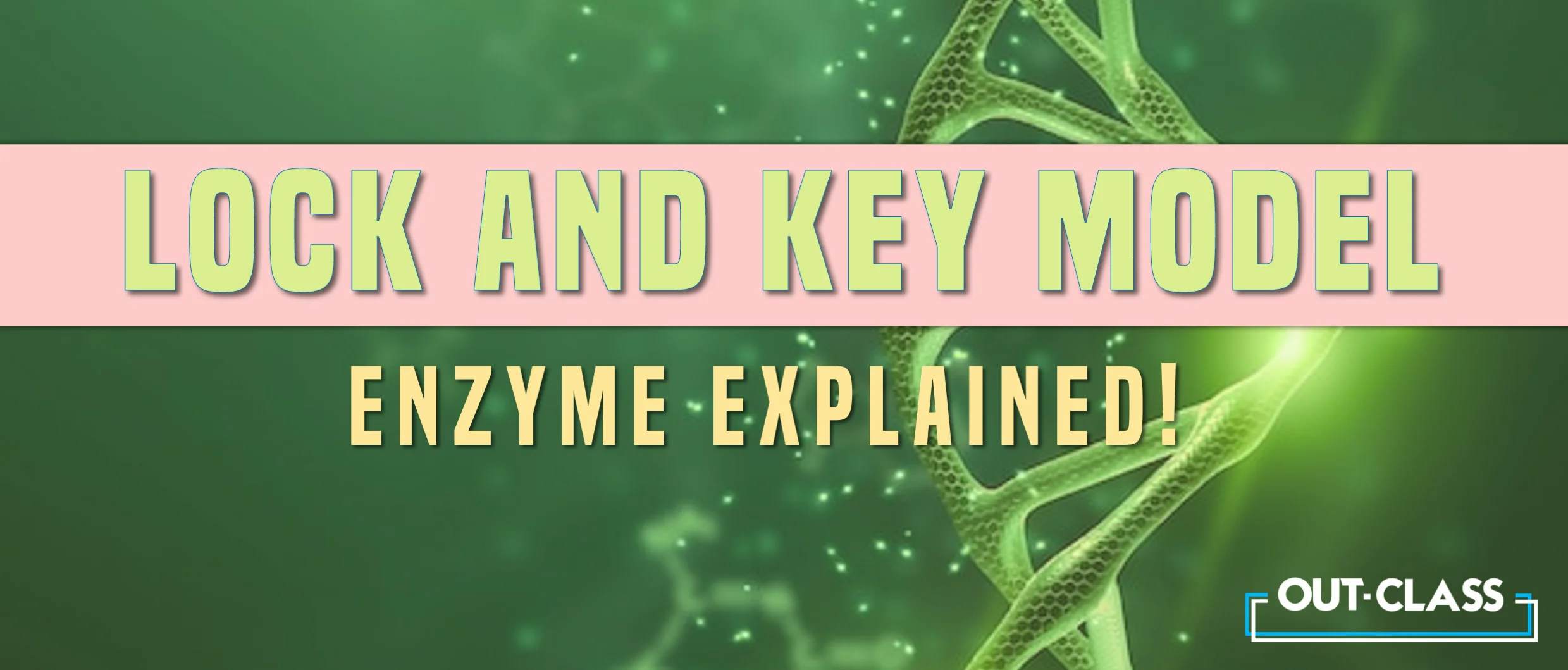
Reading about enzymes in IGCSE or O Level Biology syllabus and books might leave you confused about how they work. The “ lock and key model enzyme ” has an elegant and simple explanation. Read on below to find out.
"Just as a key fits a lock, enzymes and substrates are built for each other.”
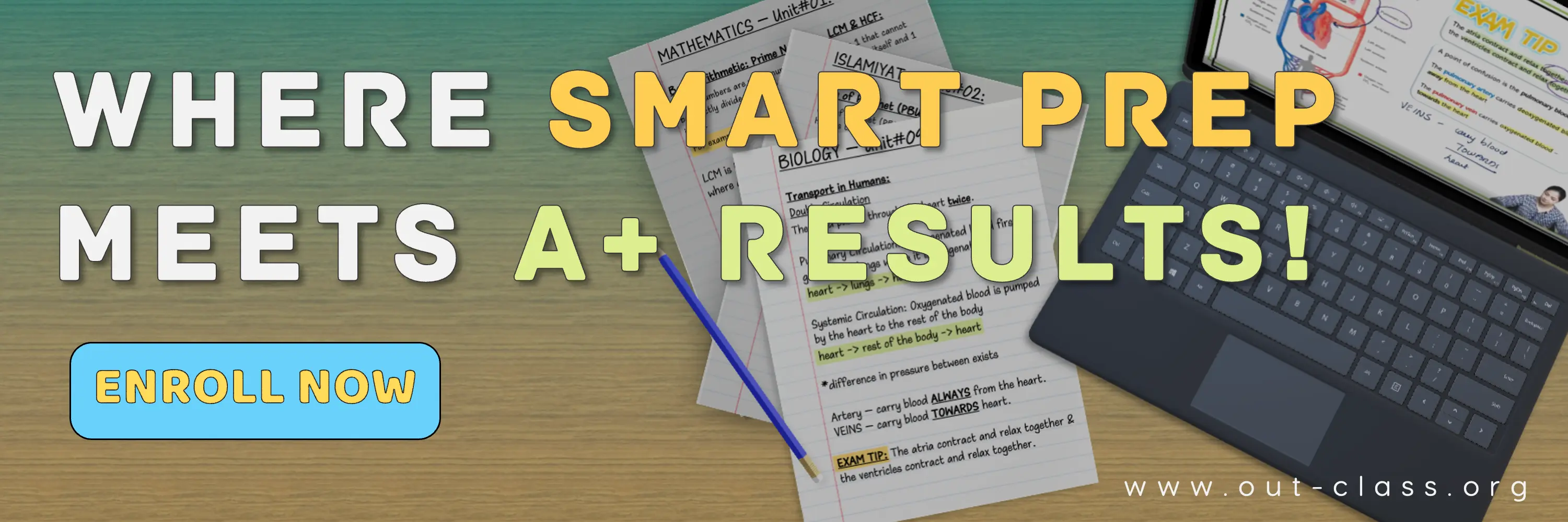
What is the Lock and Key Model?
In order to explain how enzymes work, we need to be aware of two important terms:
Enzymes: Proteins that help speed up chemical reactions .
Substrates : The chemical compounds that are processed by enzymes (e.g. they can be broken down)
Related: Enzymes
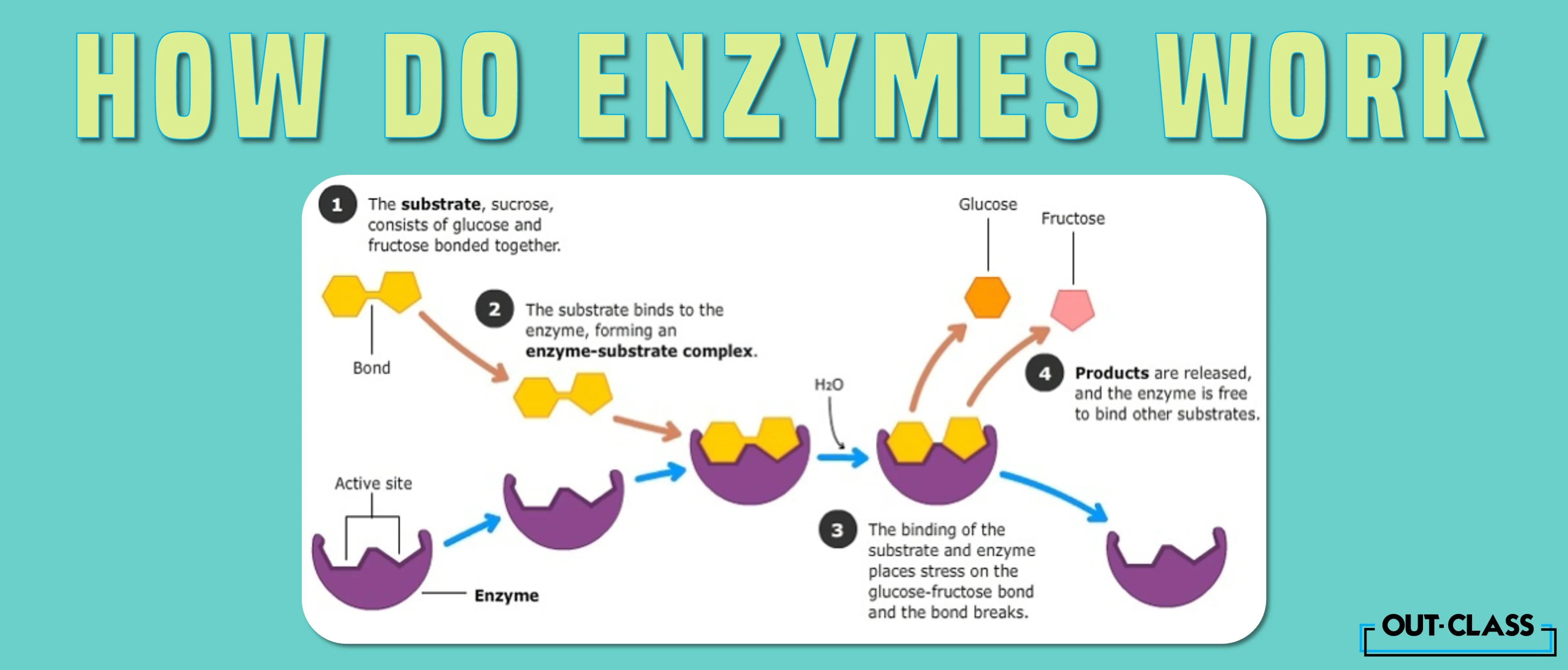
Basics of the Lock and Key Model of Enzyme Action
The basics of the lock and key model enzymes is one of the simplest models of enzyme action to understand. The lock and key model of enzyme action is similar to how we open a lock:
Only one specific key fits and opens a given lock
Similarly, only the correct substrate can fit a given enzyme, allowing it to work
*Note: This unique pairing between enzymes and substrates maintains precision in biological processes . Specific enzymes can focus on their specific reactions and this improves efficiency in maintaining cells !
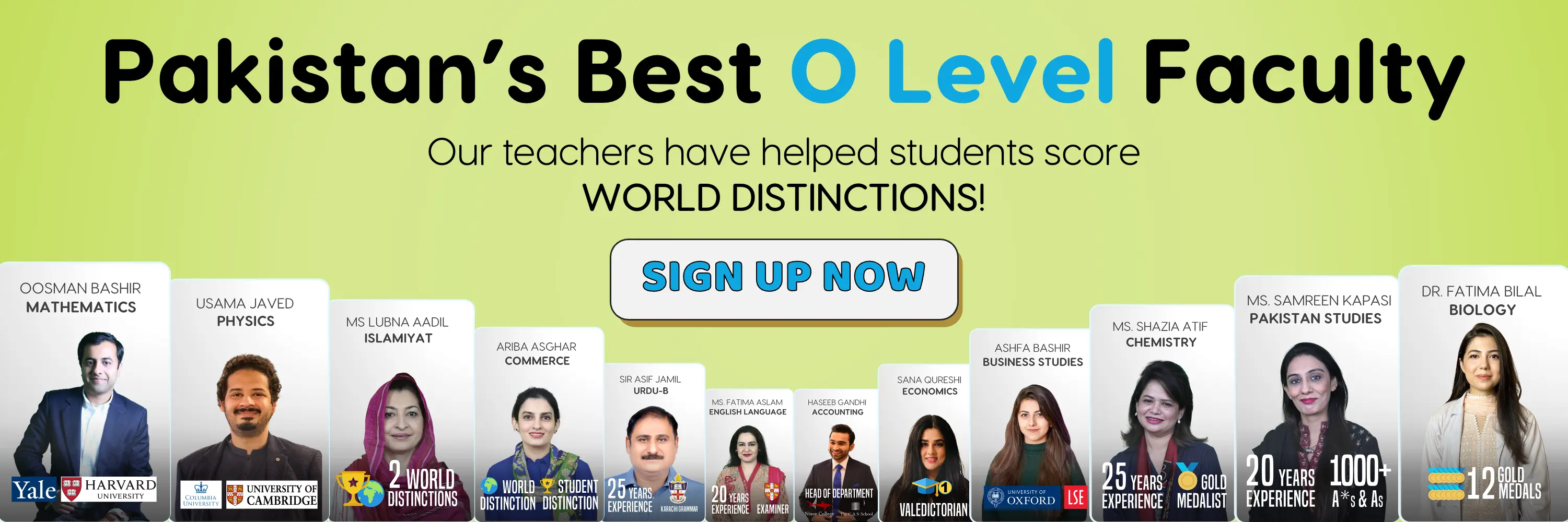
Using Lock and Key to Describe an Enzyme:
A good way to understand enzyme action is through the lock and key hypothesis:
The substrate can be thought of as a key (substrate = key)
The enzyme can be thought of as the lock (enzyme = lock)
When the substrate enters, it “ activates ” the enzyme, which starts processing the substrate
Other substrates will not be able to “activate” the enzyme, as their shape won’t match
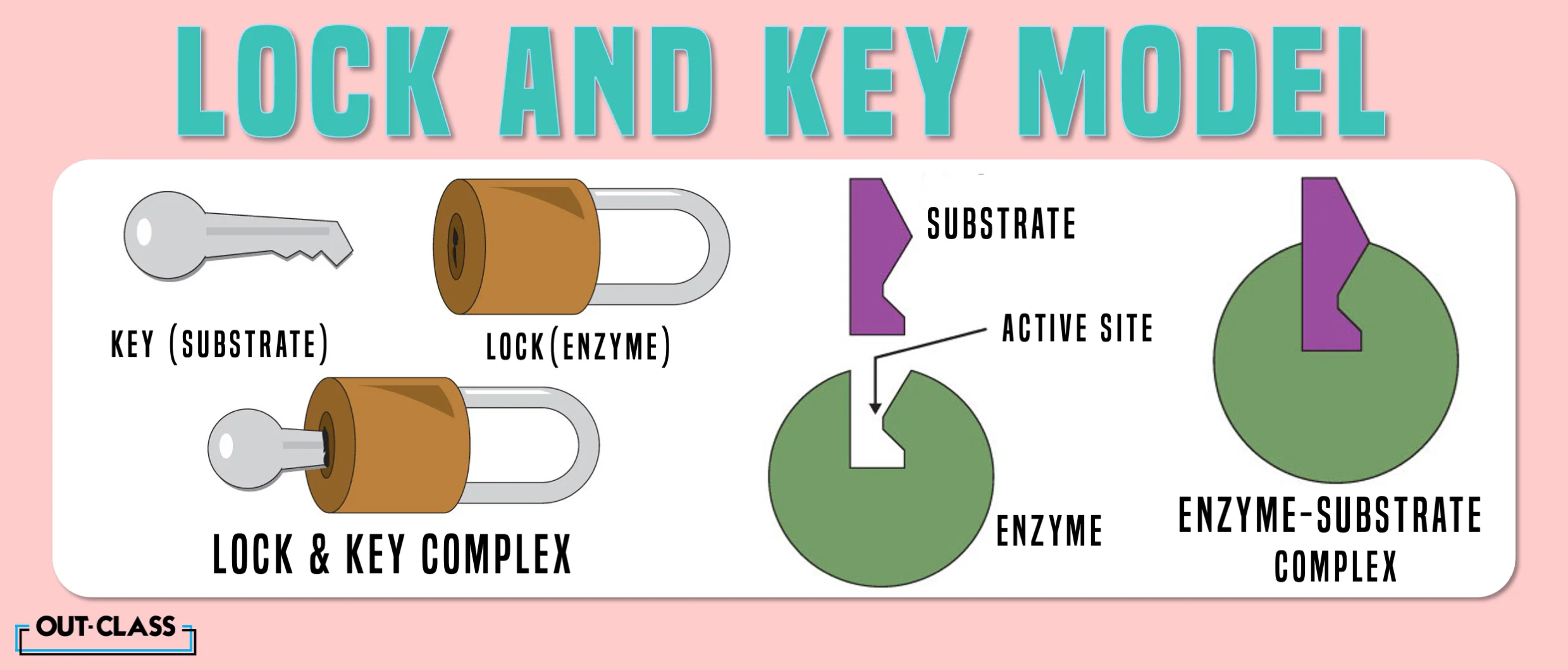
Wrapping-Up
In conclusion, enzymes speed up ( catalyze ) some key chemical reactions in organisms . The theory of lock and key model enzymes says that a given enzyme only interacts with its precise substrates.
We hope that by reading this concept guide, you developed a better understanding of O Level or IGCSE Biology . Another way to clear your concepts is by attempting and practicing with IGCSE or O Level Biology past papers .
Stay tuned to Out-Class for more study guides!
Most Common Repeated Questions:
Unlock the secrets to acing your IGCSE/O Level Biology exams with a sneak peek into the most frequently asked questions that have graced the pages of past papers!
- Explain the ‘lock and key’ hypothesis of enzyme action using a named example (5) [ Oct/Nov 2021]
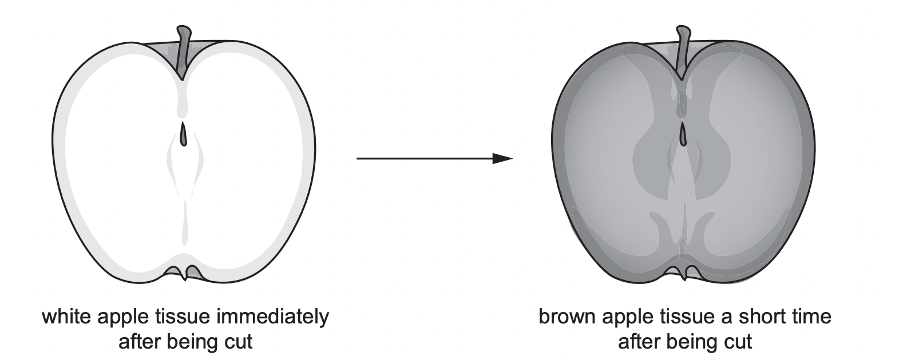
The change in colour of the apple tissue is due to a series of chemical reactions. An enzyme called PPO acts as a catalyst for one of these reactions. The colour change can be prevented by placing the cut surface of apple tissue in boiling water for a short time immediately after the fruit is cut. Explain this observation using the lock and key hypothesis of enzyme action. (4) [ Oct/Nov 2020]
Q. What are enzymes?
Enzymes are proteins that act as catalysts, speeding up chemical reactions in living organisms. They facilitate these reactions without being consumed or altered themselves.
Related: Enzymes
Q. What is the lock and key model of enzyme action?
The lock and key model is a hypothesis explaining how enzymes interact with substrates. It compares the specificity of enzyme-substrate interactions to a lock that only opens with the correct key. In this model, enzymes (locks) have specific active sites that perfectly fit their substrates (keys).
Q. How does the lock and key model maintain precision in biological processes?
The lock and key model ensures precision by allowing only specific substrates to bind with an enzyme's active site. This specificity prevents enzymes from interacting with inappropriate substrates, maintaining accuracy and efficiency in biological reactions.
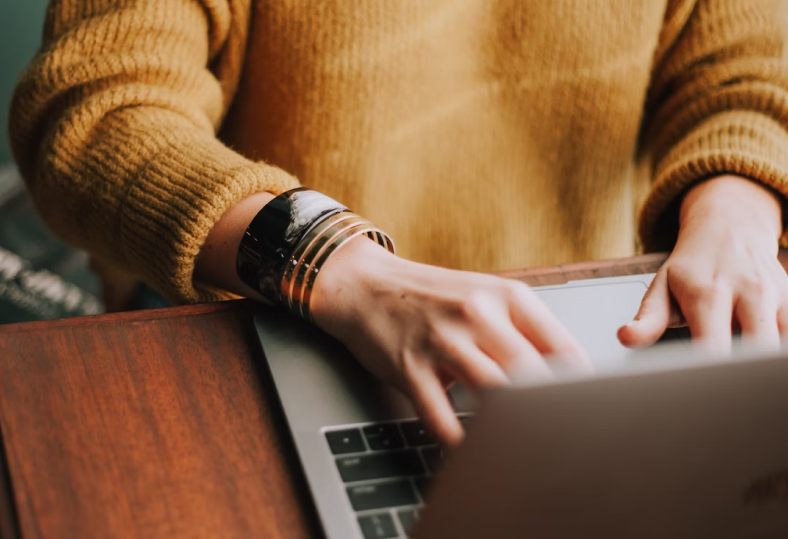
If you want to dive right in, let's start with selecting the course you want
What course are you interested in, choose from the list.

An official website of the United States government
The .gov means it’s official. Federal government websites often end in .gov or .mil. Before sharing sensitive information, make sure you’re on a federal government site.
The site is secure. The https:// ensures that you are connecting to the official website and that any information you provide is encrypted and transmitted securely.
- Publications
- Account settings
Preview improvements coming to the PMC website in October 2024. Learn More or Try it out now .
- Advanced Search
- Journal List
- HHS Author Manuscripts

Molecular Docking: From Lock and Key to Combination Lock
Ashutosh tripathi.
1 Department of Molecular and Cellular Medicine, College of Medicine, Texas A&M Health Sciences Center, College Station, Texas, USA
Vytas A Bankaitis
2 Department of Biochemistry and Biophysics, A&M Health Sciences Center, Texas, USA
3 Department of Chemistry, A&M Health Sciences Center, Texas, USA
Accurate modeling of protein ligand binding is an important step in structure-based drug design, is a useful starting point for finding new lead compounds or drug candidates. The ‘Lock and Key’ concept of protein-ligand binding has dominated descriptions of these interactions, and has been effectively translated to computational molecular docking approaches. In turn, molecular docking can reveal key elements in protein-ligand interactions-thereby enabling design of potent small molecule inhibitors directed against specific targets. However, accurate predictions of binding pose and energetic remain challenging problems. The last decade has witnessed more sophisticated molecular docking approaches to modeling protein-ligand binding and energetics. However, the complexities that confront accurate modeling of binding phenomena remain formidable. Subtle recognition and discrimination patterns governed by three-dimensional features and microenvironments of the active site play vital roles in consolidating the key intermolecular interactions that mediates ligand binding. Herein, we briefly review contemporary approaches and suggest that future approaches treat protein-ligand docking problems in the context of a ‘combination lock’ system.
Introduction
In 1894, Emil Fischer suggested that the specificity of an enzyme towards its substrate is based on the two components exhibiting complementary geometric shapes that fit perfectly like a ‘key in a lock’. This simple ‘lock and key’ analogy succinctly conceptualized the essence of enzyme substrate interaction where the ‘lock’ describes the enzyme and the ‘key’ describes the substrate or some other small molecule ligand (e.g. a small molecule inhibitor). In such systems, it is a requirement that the ‘key’ (substrate) fit appropriately into the key hole (active site/binding pocket) of the ‘lock’ (enzyme/receptor) for productive biochemistry to take place. Keys that are too small, too large, or with incorrectly positioned notches and grooves, will not fit into the lock ( Figure 1 ).

Illustration of ‘Lock and Key’ (top), Induced fit (middle) and Combination Lock (bottom) model of protein-ligand binding interaction.
But, enzymes show conformational flexibility and, on that basis, Daniel Koshland proposed a modification to the ‘lock and key’ model. Koshland’s suggestion was that active sites of enzymes are reshaped during interactions with substrate. This ‘induced fit’ model conceptualizes the ‘lock’ (enzyme) as a dynamic entity and that the ‘key’ (substrate) modulates the shape of the ‘key hole’. This concept paints a picture of an enzyme∷ligand interaction that is more akin to that of a ‘pin tumbler lock’. That is, a device where the pointed teeth and notches on the key allow the pins and wafers in the lock to move up and down until they align with the shear line of the cylindrical grooves of the key. The cylinder moves or rotates within the lock until that fit configuration is reached and the ‘lock’ opens. In an analogous manner, a ‘correct’ substrate aligns with active site residues of the enzyme to induce the appropriate conformational changes required for the desired outcome. ‘Induced fit’ is an attractive hypothesis as it accounts for why certain ligands are not substrates for an enzyme – even though they seemingly satisfy the specific shape requirements to bind to the active site ( Figure 1 ). Computational chemists are now using these basic ideas to model protein-substrate interactions. For reasons of its greater tract ability, the ‘lock and key’ paradigm has, for better or for worse, dominated the philosophical underpinnings of molecular docking approaches. In many respects, ‘induced fit’ approaches are more powerful-albeit more complicated. Below, we review these issues as these apply to molecular docking.
Molecular docking reaches for two major goals. The first is to correctly predict and identify the most favorable binding mode of a given ligand in the active site or binding pocket of a given protein. The second is to correctly rank a family of ligands in accordance to their corresponding experimentally-determined binding affinities [ 1 , 2 ]. The high-throughput version of docking, often referred to as virtual screening or in silico screening, aims to harvest small lists of potential active compounds for downstream experimental testing from a database of millions of compounds [ 3 ]. All docking protocols have two essential components: (1) a good positioning algorithm, and (2) a robust ranking or scoring system. Docking requires extensive sampling of conformational space for a ligand in the binding pocket of a protein and thereby generates large numbers of potential poses that orient a ligand within the active site. A good positioning algorithm samples ‘all’ possible binding modes, while the scoring system ranks all the solutions and identifies the most likely ‘binding mode’ of the ligand ( Figure 2 ).

Illustrates docking and scoring scheme as a two-step process. First step involves generation of poses within the binding cavity and second step involves energetic evaluation of poses to find best scoring pose that would mimic the native protein-ligand binding.
As simple as the process may sound, both components are themselves complex problems that pose significant challenges [ 4 , 5 ]. Positioning requires exhaustive exploration of accessible conformational space and binding orientations within the active site so as to extensively map interactions between active site residues and ligand. This requires that the process for generating binding modes respect a fine balance between speed and accuracy. That is, the process must not miss valuable solutions while maintaining sufficient computational efficiency to triage nonsensical binding modes. The ability to correctly score and rank the binding modes generated for a ligand presents an even bigger challenge. In cases where a number of different ligands are being interrogated, the scoring function aims to generate a rank list that corresponds to the binding affinity. This is a challenging task as many scoring functions fail to accurately predict binding affinity and often simply report a score which may or may not be at all congruent with experimentally measured binding affinities [ 6 ].
Considering the vast conformational sampling space that must often be negotiated in docking experiments, it is not computationally feasible to explore all the degrees of translational and rotational freedom of the ligand along with the internal conformational degree of freedom for protein-ligand complex. Therefore, docking experiments are typically coarse-grained so that only a restricted sampling space is covered, and a limited number of the possible binding modes are sampled. To optimize docking and scoring functions, several methods have recently been developed to add layers of sophistication to simple ‘key into lock’ ideas.
Defining the ‘Lock’
The identification and mapping of a binding site from crystal structure data can reveal key elements in protein-ligand binding [ 7 ]. Such knowledge is indispensable for docking and rational drug design since, in the majority of cases, receptor-drug interactions are specific in nature. However, this is not as trivial an undertaking as it may initially seem. The first requirement for any successful docking simulation is to define an active site or binding pocket as this is a critical step in structure-based drug design, and provides a starting point for finding new lead compounds or drug candidates [ 8 ]. A broad suite of cavity detection methods has been developed to address these issues in docking and virtual screening simulations [ 9 , 10 ].
The success of docking and structure-based design of a drug molecule for a specific target site depended largely on the quality of information regarding active site architecture because it is the size and shape of active site or binding cavity that dictates the three-dimensional geometry of ligands that will bind within. Pocket architecture also governs the directional and non-directional intermolecular interactions that mediate protein-ligand binding. Thus, clear definition of a binding pocket surface, coupled with identification of protein∷ligand interaction sites, provides a feature set for ligand orientation within a binding substructure. A target protein may have several pockets or cavities for a ligand to bind. Some might be deeply buried in the protein interior, while some might be displayed on the protein surface. However, the precise architecture of these pockets may not be absolutely clear from standard inspection of structural data as these cavities and protrusions are frequently interconnected via small and narrow channels, or are interspersed with numerous holes or voids [ 9 ]. The shape and size of binding pockets are also potentially subject to significant variations brought on by rotation of amino acid side-chains, backbone movements, loop motions, and/or ligand-induced conformational changes [ 9 ]. Fundamental uncertainties of this nature conspire to make identification of optimal dock solutions more difficult.
After defining the binding site surface, the next crucial step is to locate the interaction sites or “hot spots” within the binding site [ 11 , 12 ]. The primary goal of interaction mapping is to understand the chemical microenvironment of binding so that interaction points can be used to constrain pose possibilities and thereby restrict sampling space to a manageable size. Thus, binding site mapping is a critical step as it defines ‘lock’ parameters and sets the constraints for positioning the ligand in the defined binding region. In addition to preparing the active site for docking, the physicochemical properties and/or interaction can be represented as fields that can be mapped and visualized, interactively, in three dimensions. Using interaction maps, the spatial distributions of properties such as charge, hydrophobicity, etc. can be qualitatively analyzed [ 12 – 15 ]. Points of interaction between the ligand and active site might be elucidated and assessed qualitatively and, in some cases, semi-quantitatively. The importance of mapping interacting features is a critical endeavor since the number of ‘hot spots’ and their contributions to the larger binding process are essential for hypothesis generation. Quality interaction mapping also facilitates the docking process by defining a set of constraints that can be quantified in terms of how many, and which, interaction points might be matched by a ligand or a library of compounds. However, the harsh reality is that, even after defining the binding region for docking and extracting interaction sites, the docking process remains fraught with uncertainties that stem from the inherently dynamic physicochemical properties of the protein-ligand system.
Protein flexibility
Proteins leverage their intrinsic conformational flexibilities to carry out a wide range of biochemical processes in catalysis, protein-protein interaction and functional regulation [ 16 ]. In many cases, subtle motions in domains, flexibilities in the protein main chain, or re-orientation of side chains, changes the shape and size of the ligand binding envelope [ 17 ]. Ligand binding itself can also effect a change in the topography of binding pocket by inducing loop movements and other conformational shifts. These range from hinge movements of entire domains, to small side-chain rearrangements in residues of the binding pocket [ 18 , 19 ], and even structural transitions that involve opening/closing of otherwise rigid structural elements of the protein about flexible joints. For these reasons, it is always useful to compare holo- and apo-structures of a protein of interest whenever possible. Although most contemporary docking approaches treat ligands as flexible, it remains a challenging task to incorporate protein flexibility into the docking regime. A thorough analysis of side chain flexibility may provide invaluable insights for improving docking run and for optimizing protein-ligand interactions. Despite some recent advancements in considering protein side-chain flexibility in optimizing simulation of protein-ligand interactions, protein flexibility remains one of the most important factors in improvement of methods for docking ligands to their flexible protein partner [ 20 ].
Considering the role of water
H 2 O molecules play myriad roles in biological structure and functions. The importance of structured water molecules in biological systems cannot be overstated given their critical roles in modulating protein–ligand interactions, and these considerations take center stage in the context of drug design and discovery [ 21 ]. When a structured water molecule is displaced by a ligand and banished to “bulk” solvent, the act of displacement increases system entropy and helps drive ligand binding. That is, ligand binding is thermodynamically more favorable if the ligand displaces a tightly bound water molecule by replicating its interaction with protein [ 22 ]. For protein-ligand complexes, many water molecules are retained in the active site and contribute to the energetics of protein∷ligand interactions independent of entropic considerations. For example, waters can bridge protein and ligand and license what would otherwise represent unfavorable interactions between two chemically incompatible groups (e.g. two bases). Water molecules can also alter the “shape” and microenvironment of the active site by tightly associating with specific residues and thereby present a steric and electrostatic binding pocket profile that is different to the one presented by an anhydrous active site [ 23 , 24 ]. These varied functional involvements of water define yet another set of important considerations that must be respected in quality docking experiments and in rational design of high affinity lead molecules. Accessible surface areas of water molecules, the hydrogen bonds that involve water, the conservation and/or displacement of water, as well as the interaction energetics of water molecules are some of the factors that must be considered in docking simulations. The reality is that contemporary state-of-the art docking algorithms, and the scoring functions that accompany them, do not adequately consider all the explicit and implicit contributions of water molecules to the binding equation. Nonetheless, several docking routines include methods for identifying relevant water molecules and including those contributions in pose generation and in calculating free energies of ligand binding [ 25 ].
Protonation and ionization states of binding site residues
In addition to managing issues associated with protein flexibility and solvent, both the computational intensities and uncertainties of the docking problem are compounded for protein∷ligand systems with variable ionization states, and contributions of metals and counter ions [ 26 ]. Protein ligand interactions are sensitive to subtle changes in microenvironment of the binding site. Change in pH, buffer, ionic strength, and temperature conditions under which the data are collected also affect the microenvironment of an active site [ 27 ]. Protonation states of active site residues are typically not well-assigned, even in high resolution X-ray crystal structures, and therefore present little information to prepare the structure for docking [ 28 ]. Moreover, protein crystals are typically solvent rich (30–70%)-values that often include the crystallization buffer [ 29 ]. The accompanying ions and solvent molecules are distributed throughout the protein molecule in accord with the electrostatic properties of the solvent-accessible pockets. Altering ambient pH often alters the ionization states of residues and thereby influences the shape and electrostatic properties of the binding pocket, and ultimately the set of ligand-binding solutions [ 30 ]. Multiplicity of protonation states in ligand–protein complexes is an often overlooked aspect in protein structure preparation as emphasized by the fact that current modeling techniques frequently ignore the possibility of multiple protonation states.
There is recent progress on this front, however. New algorithms such as the computational titration protocol implemented in Hydropathic Interaction (HINT) seek to identify and optimize all possible protonation states so that rational models with atomic details can be constructed and applied to model ligand-binding energetic [ 26 , 30 , 31 ]. By modeling all ionizable residues in the binding pocket, and calculating all the possible protonation states of residues and functional groups within the active site, the computational-titration methodology realistically samples the dynamic behavior of labile H-atoms in the active site microenvironment. In particular, an important aspect of the active site microenvironment that is often ignored is the dielectric constant within the active site [ 32 , 33 ]. While comprehensive estimations of polarizability and binding energies are computationally expensive endeavors, simplified models that use macroscopic dielectric models, either uniform or distance-dependent, are being productively applied to descriptions of binding site microenvironments [ 34 , 35 ]. The message is that accurate prediction of binding free energies requires that pH, ionization and entropic contributions be taken into account in docking and virtual screening experiments.
Entropic considerations, as well as the contributions of hydrophobicity, in ligand binding cannot be overstated but are often poorly characterized and poorly quantified [ 36 , 37 ]. Entropy and hydrophobicity are difficult to measure and therefore difficult to computationally model. It is for this reason that these parameters are sacrificed in favor of computational efficiency. Most approaches consider enthalpic and entropic contributions separately and sum these interactions to a cumulative score [ 38 ]. However, protein-ligand binding is a concerted event, and entropy and hydrophobicity are thermodynamic quantities which cannot be accurately described by a simple summation. Solvation and desolvation effects that involve hydrophobic interactions are significant factors in protein∷ligand interactions but are particularly difficult to model computationally. But, the effort is worthwhile. Docking simulations that adequately consider the entropic, solvation/desolvation, and thermodynamic components of a binding reaction yield information whether the binding is enthalpy- or entropy driven and provide vital insights into the free-energy changes in the system [ 39 – 43 ].
Finding the right ‘key’
Once the ‘lock’ is defined (i.e boundary and interacting features within the binding pocket are delineated) the next core issue is to find a suitable key for the lock. To accomplish this task, the first step is fitting the ligand (key) into the binding pocket (key hole) and finding the best fit. That effort involves sampling different ligand conformations and orientations within the binding pocket and measuring the fitness of different alternative poses to identify the most favorable fit. Thus, docking approaches share two components: (i) a search algorithm that generates a sufficient set of different poses so that it exhaustively samples nearly all possible conformations and orientations for a ligand, and (ii) a scoring algorithm which evaluates the generated poses, approximates their binding energies, and identifies an optimal binding pose(s). Several different search algorithms have evolved over the past decades that were based on a variety of computational approaches [ 44 – 47 ]. Interestingly, the evolution of computational docking approaches offers interesting parallels to the evolution of thought from ‘lock and key’ to ‘induced fit’ hypotheses. Several approaches, with different degrees of sophistication, evolved from ‘rigid body’ considerations to ‘flexible ligand’ docking methods, and are still evolving into ever more sophisticated and computationally intensive ‘flexible-ligand and flexible receptor’ methods [ 48 – 51 ]. In rigid body approaches both the receptor and ligand are treated as static units and search algorithm tries to orient a rigid ligand within a rigid binding pocket [ 52 – 54 ]. Flexible-ligand methods treat the receptor (protein) as a rigid entity, but impart flexibility to the ligand and explore different conformations in systematic or random stochastic manners [ 48 – 51 , 55 ]. By contrast, ‘flexible-ligand and flexible-receptor’ approaches treat both receptor and ligand as flexible entities [ 56 – 59 ]. Despite the significant progress made in flexible protein-ligand docking, significant improvement is still needed.
One of the earliest docking approaches involved systematic search logic [ 60 , 61 ]. However, the search becomes ever more complex with increasing ligand flexibility as the number of degree of freedom of the ligand molecule obviously increases. Such an approach was implemented in methods where ligand and binding pocket were considered to be rigid and ligand was fitted using shape complementarity as determined by point complementarity or distance geometry approaches [ 62 , 63 ]. In such docking methods, the shape of both the receptor site and the ligand is interrogated based on criteria of shape and pharmacophoric points. Orientations are generated through various alignment procedures in order to maximize the pharmacophoric constraints and shape complementarity. However, it is not feasible to exhaustively explore available conformational space, and an acceptable balance has to be struck between speed and accuracy so that as many binding modes can be explored as is feasible. Fragment-based approaches that involve either incremental construction of ligand in the binding pocket, or by simply placing and joining the fragment, circumvent problems associated with combinatorial explosion of conformers generated by the previous approaches [ 64 – 66 ].
Stochastic methods involving random sampling of conformational space of ligand in the binding pocket are also being widely applied in many docking algorithms. Algorithms using Monte Carlo sampling, coupled with Metropolis criterion, are applied to exhaustively interrogate the conformational space [ 67 ]. Simulated annealing protocols, combined with grid-based energy evaluations, can be coupled with such an approach to overcome high conformational energy barriers in the sampling regime [ 68 ]. Another such stochastic approach that has been successfully implemented in docking algorithm is the genetic algorithm-based sampling of conformational space [ 69 – 71 ]. In this approach, multi-conformers referred as chromosomes are evaluated, crossed and mutated and the best possible solution is selected based on a fitness function. The ultimate solution is represented by the best scored conformation of the total conformers after a suitable number of generations. GOLD (Genetic Optimization for Ligand Docking) is the most widely used algorithm of this type for flexible molecular docking [ 72 ].
In contrast to systematic and stochastic approaches, molecular dynamics-based and heuristic tabu searches are also implemented to explore the sample space [ 73 , 74 ]. However, molecular dynamics is computationally expensive which restricts its use in docking. To circumvent the problem of exhaustive sampling, tabu search approaches are adopted where a list of already explored conformations is maintained and only unexplored spaces are sampled [ 75 ]. This avoids reinvestigating space already sampled by associating previously sampled conformations with a degree of penalty. Apart from these deterministic approaches, hybrid consensus logic combine features from other two approaches [ 76 , 77 ]. Although these approaches can exhaustively generate and sample all possible conformations within the active site, it remains a fact that the success of any docking program is measured by how well it reproduces experiment.
The success of whole molecule docking, de novo construction of molecules into a target site, or screening large virtual combinatorial libraries is ultimately dependent on the accuracy of the scoring function that ranks the compounds. Ligand orientations can be evaluated on the fly as the ligand or fragment is positioned within the cavity, or all the generated poses can be scored in the end. The scoring methods that are used in high throughput settings i.e. that deal with thousands of diverse compounds, can be evaluated by how well the corresponding relative binding affinities can be predicted. That need has spurred development of multiple methods which can be subdivided in four major approaches: force field-based methods, semi-empirical approaches, empirical scoring methods, knowledge-based potentials, and consensus scoring functions that are a combination of multiple scoring functions [ 78 – 80 ].
Force field-based methods
Force field-based scoring methods generally use a molecular mechanics force field. This parameter contains terms for intramolecular forces (e.g. bond, angle and dihedral terms) between atoms bonded to each other, plus energy terms for intermolecular forces that describe the forces between non-bonded atoms (e.g. Van der Waals and Coulombic terms). There are also a number of widely and successfully applied molecular mechanics-based scoring functions [ 81 – 84 ]. Their popularity in virtual screening programs is a reflection of their simplicity. Though faster and simpler, these functions are not ideal for simulating biomolecular interactions as those methods were developed for calculating gas phase enthalpy of binding. Thus, this class of scoring approaches has many drawbacks, primarily that these ignore hydrophobic interactions, and solvation and entropic effects.
Empirical scoring methods
Empirical scoring methods offer an alternative approach to pure molecular mechanics-based force field scoring methods [ 85 ]. The principle is that the binding free energy of a non-covalent protein-ligand complex can be factorized into a sum of localized and chemically intuitive interactions. The terms accounting for different contributions such as hydrogen bonds, hydrophobic interactions, entropic effects are normalized by weighting factors derived from regression analyses of data from training sets comprised of well characterized protein-ligand complexes. Based on the assumption of additivity, the binding affinity is estimated as a sum of interactions multiplied by weighting factors and solved by equation of the type ( 1 ):
Where fi is a simple geometrical function of the ligand (rl) and receptor (rp) coordinates [ 6 ]. However, accuracy of these methods depends upon the quality of the experimental binding data and of the crystallographic structural data of the training set.
Semi-empirical approaches
Semi-empirical scoring functions combine the above two approaches and incorporate empirical, or empirically calibrated, energetic terms for interactions that cannot be computed by pure molecular mechanics-based methods. Thus, implicit binding energy terms such as hydrogen bonding, solvent effects, hydrophobicity and entropic terms are included in the scoring functions. In contrast to force field-based scoring functions, semi-empirical scoring terms also more accurately estimate binding energies by accounting for entropic and solvation effects known to significantly affect biological interactions in aqueous medium [ 86 – 89 ].
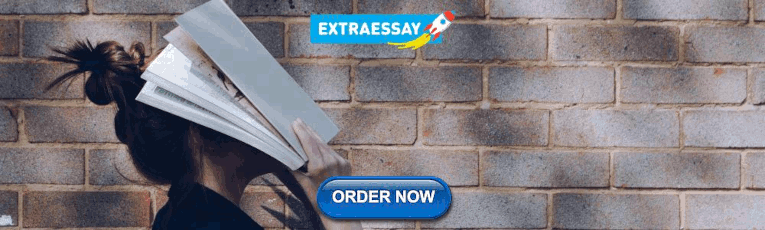
Knowledge-based scoring
Knowledge-based scoring functions [ 90 ] are rule-based regimes where rules are derived from the analysis of structural data of known and well characterized receptor-ligand interactions. The exponential growth and availability of protein-ligand crystal structures is enabling derivation and formulation of rule sets based on frequencies of chemical interactions. Scoring functions of this type seek to capture the knowledge about protein-ligand binding that is implicitly stored in the protein data bank by means of statistical analysis of structural data. That is, potentials are obtained by statistical analysis of atom-pairing frequencies observed in crystal structures of protein-ligand complexes [ 91 ]. Again, the accuracy of knowledge-based scoring function depends on the quality of experimental data, as it incorporates structural knowledge without considering inconsistencies in experimental and structural data.
Consensus scoring
Although multiple approaches have been implemented for derivation of a robust scoring function, none of the scoring functions are ideal. Invariably, various approximations are made to strike a balance between speed and accuracy. Taking into consideration the limitations of anyone scoring function, the concept of consensus scoring evolved from the base premise that a combination of different scoring functions will buffer inherent weaknesses in individual functions and offer better performance [ 92 ]. A consensus between a set of scoring functions can be reached either by averaging the rank assigned by each scoring function, or averaging the score value calculated by different functions. Ideally, the best scoring function should be able to discriminate between native and non-native binding modes and be able to calculate the actual free energy of binding.
Combination Lock and Key
Traditional docking approaches largely operate on ‘lock and key’ concepts, and this philosophy has enjoyed some successes in estimating the native binding poses of small molecule ligands. A variety of sophisticated approaches have come on-line in recent years that consider conformational flexibility for both ligand and protein [ 93 ]. However, the fact remains that both ‘lock and key’ and ‘induced fit’ approaches provide a simplistic views of ligand-binding phenomena that in actuality represent intricate molecular recognition/interaction processes. For this reason, we prefer to view protein-ligand recognition and binding reactions in terms of a ‘combination lock’ system ( Figure 1 ). In this scenario, a tandem combination of complementary features provided by both the protein and the ligand match as in case of a ‘combination lock’. Upon satisfying a suitable combination of features a binding event then ensues. For matching to occur, both feature variables on protein and ligand fine-tune and adapt in a search for the best complementarity. That is, the better the feature matching the tighter the binding. The questions then come to: (i) what are these features, (ii) how are these features encoded in the three-dimensional structure, and (iii) how is the three-dimensional feature code decoded by binding partners? The features could be geometric properties based on the three-dimensional structure of the molecule (e.g. shape, size, volume, surface area, etc.) and/or physicochemical features described by intrinsic electronic properties of a molecule (e.g. electrostatic, hydropathic and van der Waals energetic components). While the energy-based features are more dynamic in nature, and manifest themselves in three-dimensional interaction fields, the geometry-based properties are static in character. It is the sum of pharmacophoric chemical features (e.g. hydrogen bond donor/acceptors, aromatic centers, etc.), geometric features, and intrinsic electronic features of the molecules that define unique interaction fingerprints. The spatial arrangement of these various properties is a particularly discriminating property as electronic, hydropathic and van der Waals energetic properties have varying intensities in three-dimensional space and thereby form unique fields the strength of which vary from point to point and are distance dependent. The patterning of these feature sets in three-dimensional space forms the essence of molecular recognition.
Using the ‘combination lock’ concept, the essential challenge in developing the next generation of robust and predictive docking model is to accurately derive the critical interaction features and map their arrangement in three-dimensional space. These encoded features and properties must first be extracted to define exclusive ‘interaction fingerprints’ for both a ligand binding substructure on the receptor and for the ligand. These unique features and ‘interaction fingerprints’ can be stored as mathematical representations in two- or three-dimensional matrices. Subsequently, machine learning and feature matching algorithms can extract the relevant features and simulate the corresponding protein-ligand binding interactions [ 94 , 95 ]. Features extracted from physical-chemical properties and energies will have broad applicability in deriving target-focused docking and scoring in addition to developing regimes for generating target-focused libraries in silico ( Figure 3 ).

Schematic of ‘Combination Lock’ hypothesis based on feature matching. Protein and Ligand’s physicochemical properties are mapped and relevant binding features are extracted. Matching the combination of best complementary features between protein and ligand ensues optimal fit.
The availability of substantially more protein-ligand complex data and robust machine learning algorithms suggests that feature matching methodology may now be even more effective approach to predict and characterize protein-ligand binding. Recently, a combination of structure-based QSAR approach was implemented to generate descriptive and predictive models for phosphodiesterase-4 inhibitors [ 96 ]. This approach applies machine learning methodology to describes protein-ligand binding based on matching of ligand pharmacophore feature pairs with those of the target binding pocket. The method takes advantage of structure of binding pocket to derive feature sets or descriptors which is used as a reference for matching and makes it unique and target specific. Similar feature sets are generated for ligands followed by generation of structure-based pharmacophore key (SBPPK) from the protein-ligand complex based on their feature matching patterns with the binding pocket. Once the feature pairs are generated for both the receptor and ligands machine learning methods can be employed to determine pattern matches to build descriptive and predictive models of protein-ligand interactions. The method was successfully applied to study the SAR (Structure Activity Relationship) of 35 PDE-4 inhibitors. In another similar approach, atom based Interaction Fingerprint (IF) were applied to describe the patterns of ligand pharmacophores that interacted with proteins in complex [ 97 ]. These fingerprints are calculated from the distance of pairs of ligand pharmacophore features that interact with protein atoms delineating important geometrical patterns of ligand pharmacophores. From a physicochemical and pharmacological perspective, the detected patterns of ligand features would facilitate an understanding of the structure-activity relationship of the protein-ligand interactions. The method further allows a comparison of the interaction patterns of a target with those of several other targets and facilitates in sillico screening against other homologous proteins. Some of these approaches are applied as a pre-screen and to filter large databases of small molecules before they are actually docked into the protein binding pocket. This database filtering procedure was applied to virtually screen HIV protease inhibitors from ZINC database [ 98 ]. The method involved identification of binding site topology and generating site interaction points based on physicochemical property. The resultant functional/interaction properties are saved as a receptor site’s distance matrix. Similar to receptor site distance matrix, functional interaction points are located in small molecule ligand and a similar topological matrix is generated. The methodology can be seen as a comparison and matching of the ligand’s distance matrices with receptor’s matrices. Overlay and matching of receptor and ligand site matrices with each complementary pair, describes ligand’s functionalities mapped onto receptor’s binding pocket. Similar matrices can be generated for small molecules and large databases can be screened as comparing the matrices is a simple matter of matching each molecule’s distance matrix with the one generated from the protein’s binding pocket. The high proportion of known active compounds recovered in the top ranks along with target specificity signifies a promising future for the feature matching approaches for virtual screening. Such hybrid QSAR, machine learning approach that take into account ligand features as well have been applied and benchmarked against traditional rigid body docking methods and affords similar or better enrichment ratios in virtual screening [ 99 – 102 ]. We suggest that ‘combination lock’-driven approaches better capture the complex inter-relationships between feature properties of interacting biomolecules, and that implementation of such approaches will herald significant progress in our ability to model protein-ligand binding events with superior accuracy.
A primary aim of structure-based drug design is to adequately describe the binding interactions between a drug and its target. Traditionally, and perhaps in a tired analogy, protein-ligand binding is treated as a ‘Lock and Key’ system. Although pioneering studies in flexible docking and free energy calculation are making significant progress towards improving the accuracy of docking and virtual screening regimes these technologies remain complex, are time consuming and, for a variety of reasons, still suffer errors. Paradigm shifts in docking and scoring regimes are being driven by the evolution of artificial intelligence and machine learning algorithms for pose scoring and evaluation. With the availability of experimental binding data from bioactivity databases the molecular docking field is witnessing the emergence of hybrid approaches that combine ligand-based and structure-based approaches. Some of the current methods extend ligand-based machine learning strategies and principles in the direction of structure-based approaches. Based on feature extraction and correlation with crystallographic and bioactivity data, robust predictive models can now be generated complementing structure-based approach. Such hybrid ‘Combination Lock’ approaches are evolving technology and albeit with number of limitations, holds great promise for future progress in drug discovery and development.
Acknowledgments
This work was supported by grants GM44530, GM112591 from the National Institutes of Health and BE- 0017 from the Robert A. Welch Foundation (VAB). We also extend our thanks to The Laboratory for Molecular Simulation and High Performance Research Computing (HPRC) at Texas A&M University for providing software, support, and computer time.
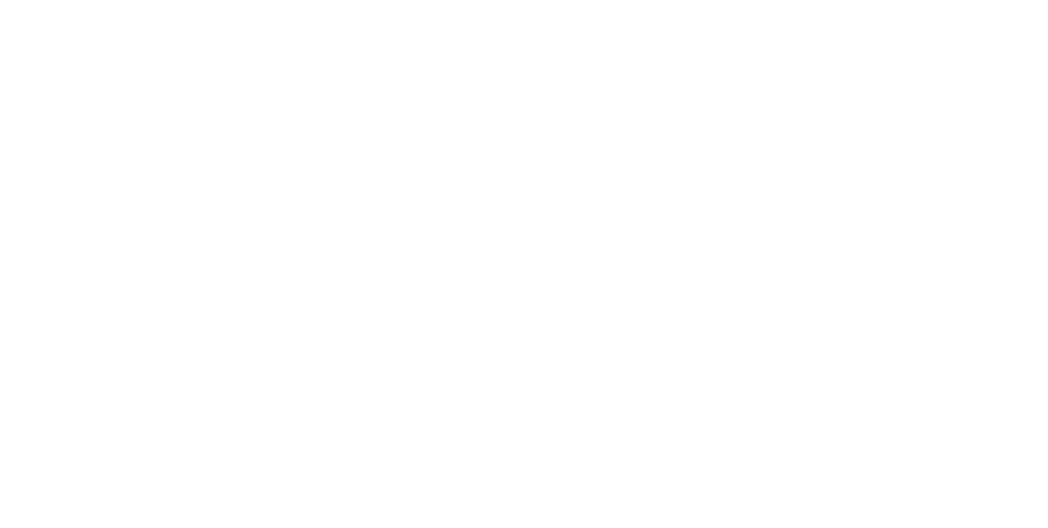
Lock and Key Model: Definition, Function, and Examples
- Post published: September 2, 2021
- Reading time: 2 mins read
Lock and Key Model Definition
A paradigm for enzyme-substrate interaction suggests the enzyme and substrate have complimentary geometric forms that fit perfectly together. Enzymes have a high level of specificity. Before they may catalyse a chemical process, they must first attach to a particular substrate.
At the moment, two models are used to describe enzyme specificity:
(1) The lock and key model
(2) The induced fit model
The enzyme-substrate interaction in the lock-and-key paradigm implies that the enzyme and the substrate have complimentary geometric forms that fit perfectly together.
Only the right size and form of the substrate (the key) would fit into the active site (the key hole) of the enzyme, similar to a key into a lock (the lock).
According to the induced fit model proposed by Daniel Koshland in 1958, the active site changes until the substrate is entirely bonded to the enzyme’s active site, at which time the final shape and charge are established.
The induced fit model, in contrast to the lock-and-key paradigm, demonstrates that enzymes are very flexible structures.
Emil Fischer proposed the lock and key model hypothesis in 1894, which demonstrates the great specificity of enzymes. It does not, however, explain the enzymes’ ability to stabilise the transition state.
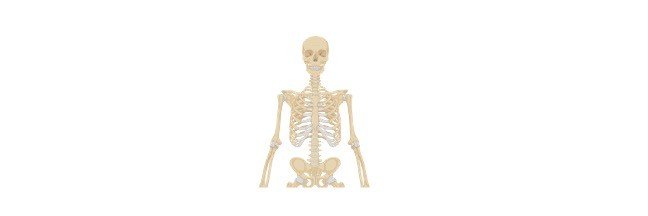
Skeletal System: Definition, Function, and Examples
Lock and key model citations.
Multivalent inhibitors for carbohydrate-processing enzymes: beyond the “lock-and-key” concept. Chemistry . 2014 Sep 8;20(37):11616-28.
Similar Post:
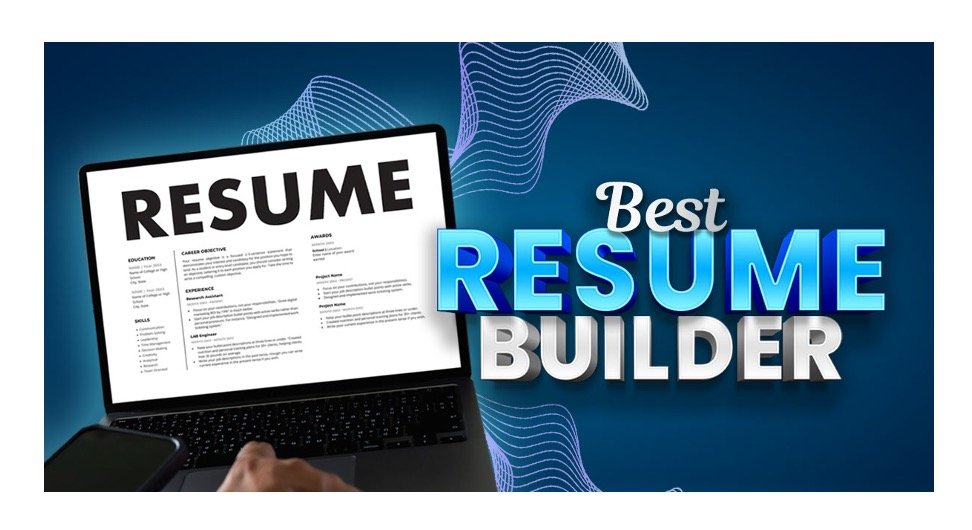
Top 10 Free Resume Builders of 2024
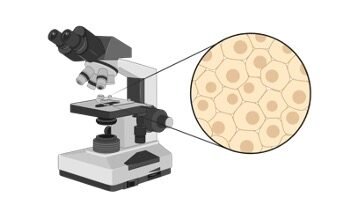
Microscope, Microscope Parts, Labeled Diagram, and Functions

26 Postdoctoral Fellowships at New York University, New York, United States
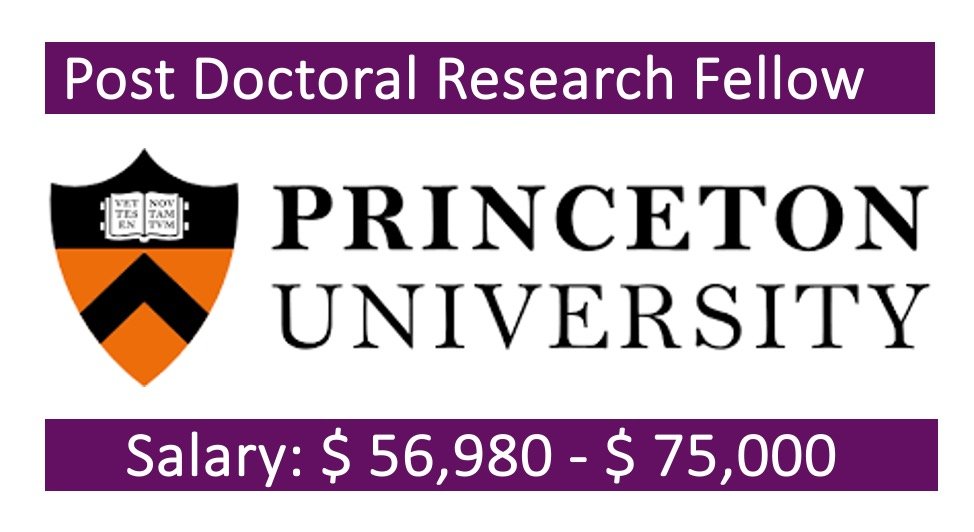
22 Postdoctoral Fellowships at Princeton University, New Jersey, United States

34 Postdoctoral Fellowships at Imperial College London, England
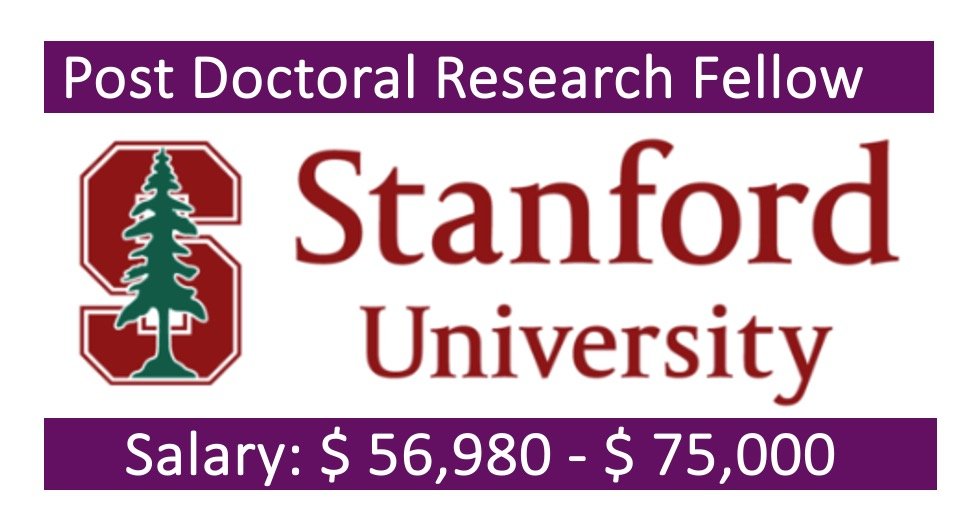
31 Postdoctoral Fellowships at Stanford University, California, United States

28 Postdoctoral Fellowships at King’s College London, England
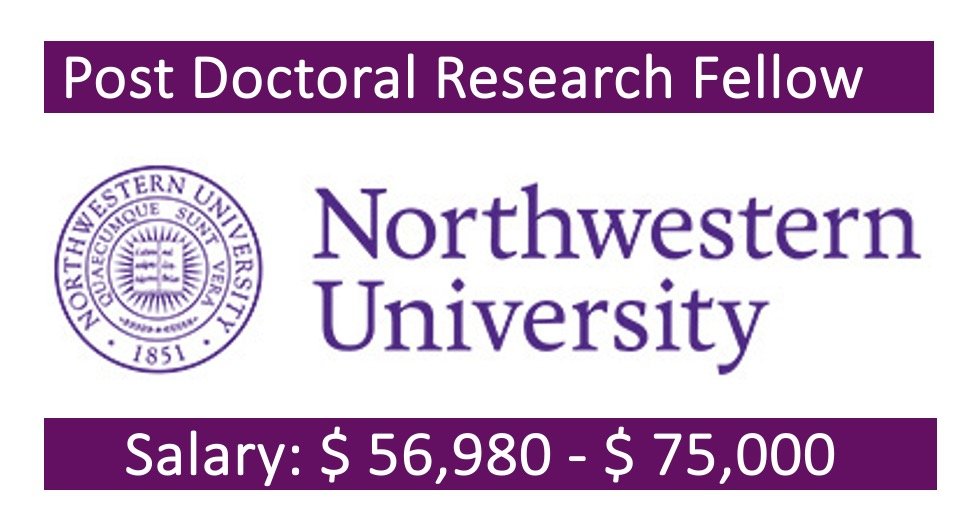
15 Postdoctoral Fellowships at Northwestern University, Illinois, United States

35 Postdoctoral Fellowships at University of Cambridge, England

23 Postdoctoral Fellowships at Moffitt Cancer Center, Florida, United States

31 Postdoctoral Fellowships at University of Edinburgh, Scotland

13 Postdoctoral Fellowships at Queen’s University Belfast, Northern Ireland
You might also like.
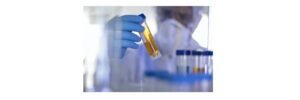
Pathogenesis and Transmission of Mycobacterium tuberculosis
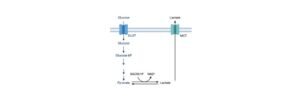
Lactate Dehydrogenase: Definition, Types, & Examples
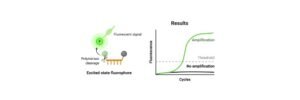
qPCR: Real-Time Polymerase Chain Reaction (RT-PCR)
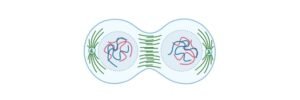
Mitosis: Phases, Diagram, Stage, and Checkpoints
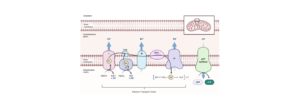
Electron Transport Chain Location
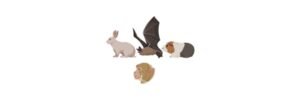
Zoology: Definition, Meaning, and Examples
Leave a reply cancel reply.
Save my name, email, and website in this browser for the next time I comment.
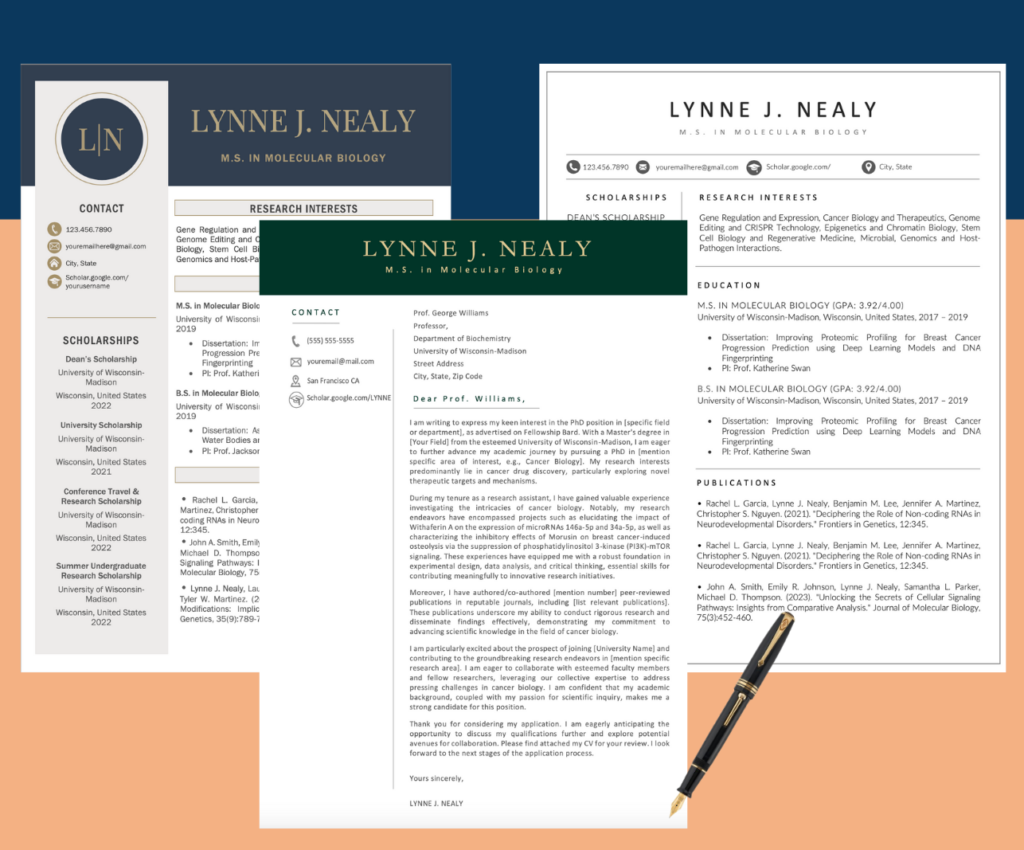
Get 3X More Success with Our Ready-to-Use Academic CV Templates!
Our CV Templates Land You in Harvard, MIT, Oxford, and Beyond!
- Subscriber Services
- For Authors
- Publications
- Archaeology
- Art & Architecture
- Bilingual dictionaries
- Classical studies
- Encyclopedias
- English Dictionaries and Thesauri
- Language reference
- Linguistics
- Media studies
- Medicine and health
- Names studies
- Performing arts
- Science and technology
- Social sciences
- Society and culture
- Overview Pages
- Subject Reference
- English Dictionaries
- Bilingual Dictionaries
Recently viewed (0)
- Save Search
- Share This Facebook LinkedIn Twitter
Related Content
Related overviews.
enzyme-substrate complex
induced-fit model
'lock-and-key theory' can also refer to...
Lock-and-key theory, more like this.
Show all results sharing these subjects:
- Life Sciences
Quick Reference
A theory to explain the mechanism of enzymatic reactions, in which it is proposed that the enzyme and substrate(s) bind temporarily to form an enzyme–substrate complex. The binding site on the enzyme is known as the ‘active site’ and is structurally complementary to the substrate(s). Thus the enzyme and substrate(s) are said to fit together as do a lock and a key.
From: lock-and-key theory in A Dictionary of Zoology »
Subjects: Science and technology — Life Sciences
Related content in Oxford Reference
Reference entries, lock‐and‐key model, lock and key model.
View all reference entries »
View all related items in Oxford Reference »
Search for: 'lock-and-key theory' in Oxford Reference »
- Oxford University Press
PRINTED FROM OXFORD REFERENCE (www.oxfordreference.com). (c) Copyright Oxford University Press, 2023. All Rights Reserved. Under the terms of the licence agreement, an individual user may print out a PDF of a single entry from a reference work in OR for personal use (for details see Privacy Policy and Legal Notice ).
date: 19 May 2024
- Cookie Policy
- Privacy Policy
- Legal Notice
- Accessibility
- [66.249.64.20|91.193.111.216]
- 91.193.111.216
Character limit 500 /500

- Table of Contents
- Random Entry
- Chronological
- Editorial Information
- About the SEP
- Editorial Board
- How to Cite the SEP
- Special Characters
- Advanced Tools
- Support the SEP
- PDFs for SEP Friends
- Make a Donation
- SEPIA for Libraries
- Entry Contents
Bibliography
Academic tools.
- Friends PDF Preview
- Author and Citation Info
- Back to Top
Locke’s Philosophy of Science
John Locke has been widely hailed for providing an epistemological foundation for the experimental science of his day, articulating the new, probabilistic form of knowledge appropriate to it. Yet, while he is in important respects a devotee of that new science, there are also significant tensions in his thought. He stands behind its experimental methods as he targets the earlier, speculative or rationalist philosophies for relying on methodologies and epistemological expectations unsuited to natural philosophy. He also frequently appears to embrace the new science’s corpuscular hypothesis, whose powers and minute particles figure prominently in his attempt to understand why we cannot hope for demonstrative certainty about natural phenomena. Still, the new science’s methodology was evolving. Just how far did Locke travel with that evolution, and what aspects of his thought prevented him from going further? As for the corpuscular hypothesis, what exactly was his stance toward it? He frequently speaks of particles and powers as if they belonged to established knowledge, and yet in explaining the hypothesis’s flaws, he seems to consider them fatal. This article will mainly emphasize the second of those related questions, though both have spurred scholarly investigation and debate.
1. Introduction
2.1 historical roots of scientia, 2.2 scientia in natural philosophy and obstacles to human attainment, 2.3 human knowledge in natural philosophy (sensitive knowledge), 3.1 tension in locke’s thought, 3.2 limitations of the corpuscular hypothesis, 3.3 main positions in the debate, 4.1 epistemology and methodology, 4.2 ontology, other internet resources, related entries.
Two features of Locke’s intellectual landscape are most salient for understanding his philosophy of science, one of which concerns the new science’s methodology, and the other its content. First, then, is the new methodological approach to understanding the natural world. This approach is accompanied by profound shifts in disciplinary boundaries and in conceptions of induction and scientific knowledge. Locke’s reaction is mostly progressive. Impressed by experimental methods and cognizant of their poor fit with the Aristotelian ideal, he defines a distinct kind of knowledge, one inferior to genuine scientific knowledge but appropriate to human sensory capacities. In so doing, he develops an epistemological basis for the new, experimental philosophy. Yet his reaction also has its conservative aspect, one that some see as having limited him in the face of the new science’s evolving methodology. He retains an ideal notion of scientific knowledge as demonstrative and certain, and while the speculative systems of the Aristotelians and the Cartesians. are the two main targets of his Essay , he shares that ideal with them.
The second salient feature is the dominant scientific theory of Locke’s day: the new science’s corpuscular hypothesis. As defined for the purposes of this article, the corpuscular hypothesis (i) takes observable bodies to be composed of material particles or corpuscles, (ii) takes impulse (action by surface impact) to be either the primary or sole means of communicating motion, and (iii) attempts to reduce colour and other qualities at the level of observable bodies to the primary or inherent properties of the particles composing those bodies. In what may be called its orthodox version (“pure mechanism,” as Ayers (1981, p. 212) calls it) the corpuscular hypothesis restricts those inherent properties to size, shape, number, and motion, and holds that all other qualities and operations are explicable in terms of that restricted set of properties. The orthodox version thus implies a proviso of contact action —that bodies causally interact only locally, by impact, such that unmediated action at a distance is deemed impossible. (Although a number of commentators use the terms ‘corpuscular hypothesis’ and ‘mechanism’ interchangeably, distinguishing them has certain benefits. For instance, it permits us to classify Isaac Newton among the corpuscularian theoristis without engaging the debate about whether he adhered to the contact action proviso. The definitions given here also agree largely with those in the entry on John Locke .) Plenist and atomist versions of the corpuscular hypothesis may be distinguished. Plenist theorists deny the void and assert a plenum of matter, as Descartes does by identifying matter with extension, and though these theorists speak of particles, their particles are not atoms, being infinitely or at least indefinitely divisible. Atomist theorists, by contrast, accept the void and take the particles or corpuscles comprising compound bodies to be indivisible, or at least probably so. Since Locke’s sympathies clearly lie with the atomist version, the term ‘corpuscular hypothesis’ shall refer to that variant throughout this article unless indicated otherwise. Locke develops central theses of the Essay in close conjunction with the corpuscular hypothesis. The most notable of these is the distinction between real and nominal essences, the former denoting a substance’s internal constitution and the latter denoting the observable properties we use to name or categorize it. Although Locke develops that distinction in connection with the primary-secondary quality distinction associated with corpuscular theorists, including his mentor, Robert Boyle, it does not follow that the two distinctions are interchangeable. Locke often treats the hypothesis with skepticism, and its status and purpose are a source of controversy.
This article examines questions connected with the two salient features noted, and in connection with the first, it also examines Locke’s relationship to Newton, a figure instrumental to the changing conceptions of scientific knowledge. Section 2 addresses questions connected to those conceptions. What does Locke take science ( scientia ) or scientific knowledge to be generally, why does he think that scientia in natural philosophy is beyond the reach of human beings, and what characterizes the conception of human knowledge that he develops for natural philosophy? Section 3 addresses the question provoked by Locke’s apparently conflicting treatments of the corpuscular hypothesis. Does he accept or defend the corpuscular hypothesis? If not, what is its role in his thought, and what explains its close connection to key theses of the Essay ? Since a scholarly debate has arisen about the status of the corpuscular hypothesis for Locke, Section 3 reviews some main positions in that debate. Section 4 considers the relationship between Locke’s thought and Newton’s. All citations of An Essay Concerning Human Understanding are indicated by ‘E’, followed by the book and section numbers. Page numbers referring to the Nidditch edition are also provided.
2. Locke on knowledge in natural philosophy: Scientia and human knowledge
Locke’s great epistemological contribution to philosophy is a conception of human knowledge suitable for the experimental science of his day, one that in natural philosophy will replace the old, Aristotelian conception. According to the Aristotelian conception, scientific knowledge— scientia —is certain knowledge of necessary truths, which can be expressed in syllogistic form, the conclusion following from self-evident premises. In the domain of natural philosophy, it is certain knowledge of real essences. Although Locke does not seriously entertain a radical skepticism—finding “a very manifest difference between dreaming of being in the Fire, and being actually in it” (E IV.ii.14, pp.537–538)—he recognizes that the demands of scientia are too stringent for the new, experimental science. Nevertheless, the concept of scientia plays an important role as he develops his conception of the probabilistic sort of knowledge that is possible for humans in the domain of natural philosophy: it serves as a foil as he explains why humans must settle for probabilistic knowledge in natural philosophy, where scientia will always remain out of reach. Scientia can serve as a foil because it is attainable for human beings in certain domains; however Locke thinks that for nobler spirits, notably angels, it is also attainable within natural philosophy.
This section begins by reviewing the history of the concept of scientia and the factors that prevent human beings from attaining it in natural philosophy, which impel Locke to develop an alternative conception of human knowledge there. This section also explains what Locke thinks scientia in natural philosophy would amount to and the lesser human knowledge that must serve us in its place.
The conception of genuine scientific knowledge that Locke inherits and to some extent retains, scientia , has its roots in Aristotle, specifically in the beginning of Book I, §2 of the Posterior Analytics . [ 1 ] For Aristotle, scientific knowledge can have only necessary truths as its objects and requires a knowledge of causes. Accordingly, scientific knowledge of a fact requires knowing its necessity by knowing its necessary relations to its causes. Although there are manifold causes, the main one at issue here is the formal cause—the nature or essence—as Aristotle indicates elsewhere. [ 2 ]
We suppose ourselves to possess unqualified scientific knowledge of a thing, as opposed to knowing it in the accidental way in which the sophist knows, when we think that we know the cause on which the fact depends, as the cause of that fact and of no other, and, further, that the fact could not be other than it is….The proper object of unqualified scientific knowledge is something which cannot be other than it is (Aristotle, Posterior Analytics , I.2).
The knower’s epistemic stance toward a necessary truth and its relation to causes is one of certainty. The certain fact is demonstrable via a syllogism in which the premises are self-evident, requiring no demonstration themselves.
We do know by demonstration. By demonstration I mean a syllogism productive of scientific knowledge, a syllogism….The premisses must be primary and indemonstrable; otherwise they will require demonstration in order to be known, since to have knowledge, if it be not accidental knowledge, of things which are demonstrable, means precisely to have a demonstration of them. The premisses must be the causes of the conclusion, better known than it, and prior to it; its causes, since we possess scientific knowledge of a thing only when we know its cause; prior, in order to be causes; antecedently known, this antecedent knowledge being not our mere understanding of the meaning, but knowledge of the fact as well (Aristotle, Posterior Analytics , I.2).
The stipulation that the premises of a scientific demonstration must be indemonstrable, that is, self-evident, leads to a prima facie difficulty. This conception of scientific knowledge is intended to encompass not only conceptual propositions, but also propositions about the real natures or essences of substances, which are propositions about the world. As with any other demonstration, a demonstration in natural philosophy must have premises that are self-evident, since otherwise a regress would ensue. Propositions about the world are experience-based, however, and therefore, it is not clear how a demonstration’s premises could be self-evident.
It would be overstating matters to say that this problem appears only from a contemporary perspective; there was some recognition of it in the ancient and medieval periods. [ 3 ] Still, before the advent of the experimental science, the problem was not strongly felt because experience was understood in a different way. For one thing, the notion of an experiment—an artificially constructed, single event or series of events designed to test for a predicted outcome—did not exist. Moreover, among the scholastic Aristotelians, a single, naturally occurring event could not by itself be regarded as revealing of natural processes; such an event could be a “monster,” that is, an event that conflicts with nature rather than having been produced by it. [ 4 ] Events as generally experienced, however, were regarded as being revealing of nature and consequently, they could provide the universal truths needed as premises in the syllogism. [ 5 ] How was the gap between events as usually experienced—which still amounts to a limited sample of evidence—and the universal claim derived from them bridged? It is only from the modern and contemporary perspectives that such a gap exists to be bridged. For Aristotle and medieval thinkers, human faculties are so constituted as to be able to apprehend nature, that is, to discern the essences of substances. [ 6 ] In short, then, because the internal essences that form the content of natural philosophy are real, and because our faculties are constituted to apprehend those real essences, natural philosophy can be a science; it is a domain in which certain, demonstrative knowledge can be had, despite its dependence upon experience.
The exemplars of scientific knowledge, to be sure, are conceptual disciplines, not only geometry, but also rational theology, the latter being the quintessential science for the medievals. [ 7 ] Yet for the most part, natural philosophy stands side by side with conceptual disciplines, even into the modern period. Bacon, though associated with induction, accepts the demonstrative conception of scientific knowledge, and so does Galileo, who uses experiments to reveal fundamental principles (though also using them in other ways). [ 8 ] As empirical methods are refined and more widely applied, however, the belief that natural philosophy can stand under the umbrella of scientia comes under increasing pressure. [ 9 ] Some thinkers resist the pressure, most notably Descartes, who derives his laws of nature by an a priori reflection upon God’s nature, and, placing his confidence in these rationalist methods, denies that his third law of nature is undermined when observations of colliding bodies conflict with it. [ 10 ] Yet for the experimentalists themselves, including Locke’s mentor, Boyle, observations and experiments are the primary route to knowledge. (It should be noted, however, that most advocates of experimentalism did not consider speculation wholly illegitimate, instead insisting that it be delayed until considerable experimental and observational evidence had been amassed; see Anstey (2011, pp. 4,5).) It is this approach that puts natural philosophy on the path to Hume’s problem of induction and most influences Locke. [ 11 ]
As indicated earlier, scientia serves as the backdrop against which Locke develops the conception of the type of knowledge that is humanly possible in natural philosophy. Having retained the ideal of scientia exemplified by geometry while also having assimilated the significance of Boyle’s experimental method, Locke is driven to his characteristic pessimism about the kind and extent of knowledge possible for us in natural philosophy. “The meanest, and most obvious Things that come in our way, have dark sides, that the quickest Sight cannot penetrate into” (E IV.iii.22, p. 553). Due to the weakness of our faculties, Locke suspects, “natural Philosophy is not capable of being made a Science” (E IV.xii.10, p. 645). This section considers Locke’s general notion of scientia , what would be required for scientia in natural philosophy, and the obstacles that prevent human beings from attaining scientia in that domain.
2.2.1 Scientia in general
Locke takes knowledge generally to consist in “the perception of the connexion and agreement, or disagreement and repugnancy of any of our Ideas” (E IV.i.1–2, p. 525), and among the three kinds of knowledge that he distinguishes—intuitive, demonstrative, and sensitive—the former two are kinds of certain knowledge. Intuitive and demonstrative knowledge differ in the number of intuitions involved, and consequently, differ in their degree of certainty (E IV.ii.14, pp.537–538). Intuitive knowledge is the most certain because the truth is grasped immediately. There are no intermediate steps, and doubt is impossible because the mind can no more avoid recognizing the truth than the open, functioning eye could avoid seeing light when turned toward the sun (see E IV.ii.1, p. 531). Demonstrative knowledge, though also qualifying as certain, is less so because it involves intermediate steps. We cannot grasp immediately that the three angles of any triangle are equal to two right triangles but must instead construct the steps of a proof. Upon doing so and grasping the connections among the proof’s steps, we have demonstrative knowledge (E IV.ii.2–3, p.531–532). Intuitive and demonstrative knowledge are forms of scientia , then, which Locke defines as “certain universal Knowledge” (E IV.iii.29, p. 559). Mere “particular matters of fact” (E IV.iii.25, pp. 555–56) do not qualify.
To understand Locke’s notion of scientia , we must consider its objects: real essences and the necessary connections that flow from them. According to the Aristotelian view, a single essence both grounds the properties of a thing, making it what it is, and provides the basis for classifying it. Repudiating that view, Locke draws a distinction between real and nominal essences. [ 12 ] Whereas the nominal essence consists of the set of observable qualities we use to classify a thing (which implies that the nominal essence could vary across time or communities), the real essence (or real or internal constitution, as he sometimes writes) is that which makes a thing what it is.
Essence may be taken for the being of any thing, whereby it is what it is. And thus the real internal, but generally in Substances, unknown Constitution of Things, whereon their discoverable Qualities depend, may be called their Essence . This is the proper original signification of the Word, as is evident from the formation of it; Essentia , in its primary notation signifying properly Being . And in this sense it is still used, when we speak of the Essence of particular things, without giving them any Name (E III.iii.15, p. 417).
Discussion of real essence often focuses upon the real essences of material substances, and in that case we say that the real essence is the causal ground of the substance’s perceivable properties (for further details and a useful chart, see the entry by Jones, “Locke on Real Essence”). As will be discussed in a subsequent section, many commentators interpret Locke as identifying a material substance’s real essence with some subset of its constituent corpuscles’ primary qualities, but that identification presums Locke’s acceptance of the corpuscular hypothesis (e.g., Osler 1970, p. 12; Mandelbaum 1964, p.1). According to another interpretation, the real-nominal essence distinction is metaphysical, and thus more fundamental than the primary-secondary quality distinction, which is a physical distinction, belonging as it does to a particular physical theory, the corpuscular hypothesis. Yet leaving that debate aside for the moment, we may note from the first sentence of the above-quoted passage that Locke does not restrict the notion of real essence to substances. This means that we can speak of, say, the real essence of a triangle, understanding it as that which grounds the triangle’s qualities, making it what it is.
Since a substance’s qualities flow from its real essence, having scientific knowledge of a substance requires knowing both its real essence and the necessary connections between that and its other qualities. Geometry serves as an exemplar, as it did for so many of Locke’s predecessors. In knowing what a triangle is, we cannot conceive things being otherwise than that the sum of its three angles equals the sum of two right angles.
Such knowledge is so certain that we cannot conceive even of God having made things otherwise:
Thus the Idea of a right-line Triangle necessarily carries with it an equality of its Angles to two right ones. Nor can we conceive this Relation, this connexion of these two Ideas , to be possibly mutable, or to depend on any arbitrary Power, which of choice made it thus, or could make it otherwise (E IV.iii.29, pp. 559–560).
Scientia is possible in another conceptual domain also: morality. Morality is characterized by discernible necessary connections, and Locke is adamant that we can have the same level of certainty there as in geometry.
Where there is no Property, there is no Injustice , is a Proposition as certain as any Demonstration in Euclid : For the Idea of Property , being a right to any thing; and the Idea to which the Name Injustice is given, being the Invasion or Violation of that right; it is evident, that…I can as certainly know this Proposition to be true, as that a Triangle has three Angles equal to two right ones (E IV.iii.18, pp. 549–50).
2.2.2 Scientia in natural philosophy
What would be required for scientia in natural philosophy? Since scientia generally concerns real essences, and since natural philosophy for Locke concerns material substances and their powers, scientia in natural philosophy would be knowledge of material substances’ real essences and their necessary connections to the qualities flowing from them.
If we could have scientia in natural philosophy, we could know a substance’s qualities without making observations or experiments. To take one of Locke’s frequent examples, if we could know gold’s real essence, we would then know its qualities, even if not a single sample of gold existed.
Had we such Ideas of Substances, as to know what real Constitutions produce those sensible Qualities we find in them, and how those Qualities flowed from thence, we could, by the specifick Ideas of their real Essences in our own Minds, more certainly find out their Properties, and discover what Qualities they had, or had not, than we can now by our Senses: and to know the Properties of Gold , it would be no more necessary, that Gold should exist, and that we should make Experiments upon it, than it is necessary for the knowing the Properties of a Triangle, that a Triangle should exist in any Matter, the Idea in our Minds would serve for the one, as well as the other (E IV.vi.11, p. 585).
Which qualities exactly would we be able to deduce? We would be able to deduce a substance’s tertiary qualities, that is, its powers to produce certain effects in other substances. [ 13 ] If we knew the real essences of opium and hemlock, then just as if we were performing a geometric deduction, or just as a locksmith understands why a given key will open one lock rather than another, we could deduce that opium produces sleep, that hemlock causes death and why each substance produces its effects.
I doubt not but if we could discover the Figure, Size, Texture, and Motion of the minute Constituent parts of any two Bodies, we should know without Trial several of the Operations one upon another, as we do now the Properties of a Square, or a Triangle. Did we know the Mechanical affections of the Particles of Rhubarb, Hemlock, Opium , and a Man , as a Watchmaker does those of a Watch, whereby it performs its Operations, and of a File which by rubbing on them will alter the Figure of any of the Wheels, we should be able to tell before Hand, that Rhubarb will purge, Hemlock kill, and Opium make a Man sleep….The dissolving of Silver in aqua fortis , and Gold in aqua Regia , and not vice versa , would be then, perhaps, no more difficult to know, that it is to a Smith to understand, why the turning of one Key will open a Lock, and not the turning of another (E IV.iii.25, pp. 555–56) (cf. Boyle, who had had the same idea, explaining it at length in The Origin of Forms and Qualities , 1666, pp. 16–19).
Knowing real essences would enable us to deduce tertiary qualities, then. What about secondary qualities, however? Here, matters are initially less clear; Locke seems to be saying that while having more acute senses would not eliminate the secondary quality of sound, but might do away with the secondary quality of color. In a passage where he imagines our having very acute senses, including “microscopical eyes,” he unquestionably assumes that we would still experience the secondary quality of sound: “If our Sense of Hearing were but 1000 times quicker than it is, how would a perpetual noise distract us” (E II.xxiii.12, pp. 302–303). Yet, in a preceding passage, he suggested that if our faculties were designed for detecting real essences, we would not experience color at all:
Had we Senses acute enough to discern the minute particles of Bodies, and the real Constitution on which their sensible Qualities depend, I doubt not but they would produce quite different Ideas in us; and that which is now the yellow Colour of Gold, would disappear, and instead of it we should see an admirable Texture of parts of a certain Size and Figure. This Microscopes plainly discover to us: for what to our naked Eyes produces a certain Colour, is by thus augmenting the acuteness of our Senses, discovered to be quite a different thing; and the thus altering, as it were, the proportion of the Bulk of the minute parts of a coloured Object to our usual Sight, produces different Ideas , from what it did before….Blood to the naked Eye appears all red; but by a good Microscope, wherein its lesser parts appear, shews only some few Globules of Red, swimming in a pellucid Liquor; and how these red Globules would appear, if Glasses could be found, that yet could magnify them 1000 or 10000 times more, is uncertain (E II.xxiii.11, pp. 301–302).
Reflecting upon these examples, however, suggests that he is not, or not always, imagining that microscopical eyes would do away with color altogether; rather, it might in some cases enable us to view tinier particles having different colors than those we perceive in the aggregate object. In his example, only as an aggregate body does blood as an aggregate body appears uniformly red; under a microscope, only the globules appear red, while some other parts of it seem translucent. Once the microscope is used, color is not eliminated from the experience of seeing blood, but is instead seen as being differently distributed. A pixelated painting provides a rough analogy; a shape seen from afar may appear uniformly green, but up close is seen as comprising tiny blue and yellow dots.
2.2.3 Obstacles to human attainment
Scientia in natural philosophy would require knowledge of both real essences and their necessary connections among qualities, yet neither is possible for human beings, Locke concludes. One obstacle to scientia , then, is that real essences escape us. God has given us sensory capacities that are suitable for such practical endeavors as finding our way to the “market and exchange,” but as the “microscopical eyes” passage indicates, they are not useful for detecting the minute parts of bodies.
Another obstacle is that we are almost entirely unable to discern the necessary causal connections among the qualities of substances. (And Locke does take those connections to be necessary—but does he construe them in terms of nomological or logical necessity? Given his view that demonstrative knowledge in natural philosophy is possible for immortals and serves as an ideal for mortals, he seems to be thinking in terms of logical necessity, much like the Aristotelians he was reacting against (see Ott, 2009, p. 13). Locke does find two instances in which we can discern necessary connections between qualities of bodies: “Some few of the primary Qualities have a necessary dependence, and visible connexion one with another, as Figure necessarily presupposes Extension, receiving or communicating Motion by impulse, supposes Solidity” (E IV.iii.14, p. 546). Apart from these two exceptions, however, necessary connections escape us. In part, this is due to the first obstacle, our inability to discover real essences, due to the minuteness of particles. It is also due, however, to the remoteness of so many bodies, those which lie “beyond this our Earth and Atmosphere…even beyond the Sun, or remotest Star our Eyes have yet discovered” (E IV.vi.11, p.586–87, and IV.vi.12, p.587). For according to Locke’s speculations, all things might be causally interconnected in complex ways, in which case knowing one real essence would require knowing all those with which it is causally connected.
Still, while human beings cannot attain scientia in natural philosophy, there are other epistemic agents who can. God certainly knows real essences (E III.vi.3, p. 440), and “’tis possible Angels have” ideas of real essences as well (E III.vi.3, p. 440).
It be not to be doubted, that Spirits of a higher rank than those immersed in Flesh, may have as clear Ideas of the radical Constitution of Substances, as we have of a Triangle, and so perceive how all their Properties and Operations flow from thence, but the manner how they come by that Knowledge, exceeds our Conceptions (E III.xi.22, p. 520).
That Locke finds it natural to speak in the same breath of matter and spirits marks him as belonging to the age of natural philosophy rather than science. It is because knowledge of necessary connections can be referred to these higher epistemic agents that scientia is so strongly entrenched as an ideal, even though he recognizes the need for a quite different conception of knowledge.
The conclusion that no intuitive or demonstrative knowledge of substances is possible for us because their real essences and necessary connections remain out of reach leaves Locke at a crossroads. One path is the skeptical belief that without certainty, no knowledge of substances is possible at all. He rejects that path, denying that hyperbolic doubt could be genuine for either the self (E IV.ix.2, pp. 619–20) or for external objects (E IV.xi.3, p. 631). The other path is the one that he follows. Here, he lowers the bar by admitting a third kind of knowledge, which lacks certainty: sensitive knowledge. [ 14 ]
Sensitive knowledge is knowledge of the “effects [that] come every day within the notice of our Senses,” without an understanding of their causes; “we must be content to be ignorant of” those causes (E IV.iii.29, pp. 559–560). Instead of knowing real essences, the causal basis of the properties we perceive, we know only those perceived properties, from which we construct nominal essences. Instead of employing deduction, we are forced to rely upon “trials”—observations and induction. Instead of knowing the necessary connections holding between a substance’s real essence and its other qualities, including its tertiary qualities (which might include, recall, causal connections with substances beyond the remotest star), we know only the co-existences of properties. And from the mere, regular co-existence of properties found in observed cases, Locke observes, we could not know with certainty that the same set will be found co-existing in the next case.
For all the Qualities that are co-existent in any Subject, without this dependence and evident connexion of their Ideas one with another, we cannot know certainly any two to co-exist any farther, than Experience, by our Senses, informs us. Thus though we see the yellow Colour, and upon trial find the Weight, Malleableness, Fusibility, and Fixedness, that are united in a piece of Gold; yet because no one of these Ideas has any evident dependence , or necessary connexion with the other, we cannot certainly know, that where any four of these are, the fifth will be there also, how highly probable soever it may be (E IV.iii.14, p. 546).
Our discoveries about co-existing properties—are merely contingent particulars, or, insofar as they are applied beyond the particular cases we have actually observed, are mere probability. Nevertheless, they can qualify as real knowledge. To qualify, our ideas must meet certain conditions. The complex idea that we refer to as a substance must comprise all and only those simple ideas that we have found to co-exist in nature. With this, Locke aims to show that sensitive knowledge deserves its appellation, since it can be distinguished from arbitrary or otherwise poorly grounded claims (e.g., that fluidity has been found to co-exist with brittleness, in a single substance and at a single given temperature. [ 15 ] ) Sensitive knowledge is something far less than scientia but far more than ungrounded opinion.
Herein therefore is founded the reality of our Knowledge concerning Substances , that all our complex Ideas of them must be such, and such only, as are made up of such simple ones, as have been discovered to co-exist in Nature. And our Ideas being thus true, though not, perhaps, very exact Copies, are yet the Subjects of real (as far as we have any) Knowledge of them (E IV.iv.12, p. 568).
As for general claims about substances based upon observed particular matters of fact, these too can qualify as real knowledge. Admittedly, when four of the five properties previously found co-existing together occur again, it is only probable that the fifth will be present as well. Yet we can still form an abstract idea of gold, as a substance having all five properties, and call this general claim knowledge because “whatever have once had an union in Nature, may be united again” (E IV.iv.12, p. 568).
Has contemporary science enabled us to go beyond sensitive knowledge? In particular, have discoveries about compounds, elements, and subatomic particles provided us with knowledge of real essences? Much of the force of this question derives, to paraphrase Nicholas Jolley, from the fact that many of those discoveries about matter’s structure were not conceived empirically, but only confirmed empirically; they were initially conceived as possibilities through the hypothetico-deductive model, and the predictions deduced from the models were then compared to empirical data (Jolley 2002, p. 69). Yet as Jolley also points out, these commentators may have missed the full import of Locke’s geometric model; in a passage quoted earlier, Locke tells us explicitly that if we knew the real essence of gold, we could deduce its qualities even if the metal did not exist. [ 16 ] So while the predictions of any model developed via the hypothetico-deductive model must survive the test of observations, observations in Locke’s scientia are wholly unnecessary . To put the point another way, Locke takes natural philosophy to be an empirical domain only for human beings, whose faculties are impoverished. For nobler spirits, it would resemble geometry.
3. Tension in Locke’s thought and a consequent debate
The last few decades have seen a lively debate about the role of the corpuscular hypothesis in Locke’s Essay . This section examines the sources of that debate and reviews some of the main positions figuring in it.
As we have seen, Locke develops some central theses of his Essay in connection with the corpuscular hypothesis. In his theory of ideas, corpuscles provide at least a structural basis for simple ideas, and depending upon one’s interpretation, there may be a causal relationship as well. Further, and of particular interest here, Locke often appears to identify a material substance’s real essence with the set or some subset of its component particles’ primary qualities. In the following well-known passage, for instance, he points to the primary qualities of a body’s parts—their bulk or solidity, motion, and shape—as the causal ground of the qualities we perceive.
The particular Bulk, Number, Figure, and Motion of the parts of Fire, or Snow, are really in them whether any ones Senses perceive them or no: and therefore they may be called real Qualities , because they really exist in those Bodies. But Light, Heat, Whiteness , or Coldness, are no more really in them, than Sickness or Pain is in Manna. Take away the Sensation of them; let not the Eyes see Light, or Colours, nor the Ears hear Sounds; let the Palate not Taste, nor the Nose Smell, and all Colours, Tastes, Odors, and Sounds, as they are such particular Ideas , vanish and cease, and are reduced to their Causes, i.e. Bulk, Figure, and Motion of Parts (E II.viii.17, pp. 137–138).
He similarly seems to identify the real essence of bodies with primary qualities just prior to the “microscopical eyes” passage. There, he suggests that instead of seeing colors (or instead of seeing them as we currently do), we could discover bodies’ internal constitutions, if only we knew the “texture and motion of the minute Parts of corporeal things” (E II.xxiii.12, pp. 302–303). A commitment to the corpuscular hypothesis is again suggested when he despairs of understanding the production of secondary qualities: even if “we could discover the size, figure, or motion of those invisible parts, which immediately produce them [secondary qualities],” we still cannot discover any “undoubted Rules” concerning their production or connection, nor “conceive how any size, figure, or motion of any Particles, can possibly produce in us the Idea of any Colour, Taste , or Sound ” (E IV.iii.13, p. 545). Here, he appears to despair of understanding how secondary qualities are produced by primary ones; he appears to take the corpuscular hypothesis’s reductionist claim to be true, but he despairs that we could understand how the reduction works.
His discussion of tertiary qualities is similar. If we knew the “Figure, Size, Texture, and Motion of the minute Constituent parts of any two Bodies,” we would then be able to derive tertiary qualities; we would be able to deduce that opium causes sleep, and we would understand why (E IV.iii.25, pp. 555–56; see also E IV.iii.13, p. 545). In all of these passages, then, and in many similar ones, Locke appears to accept at least some components of the corpuscular hypothesis—that material bodies are compounded from minute particles, and certain observable qualities are reducible to the particles’ primary qualities of size, shape, and motion. This tendency to speak as though the corpuscular hypothesis is true, either in whole or in part, has been termed Locke’s “dogmatic” side (Downing 2007).
In apparent tension with this so-called dogmatic side is what has been termed his “agnostic” or “skeptical” side. The following features of his discussion seem to suggest that he has reasons either for remaining agnostic about whether the corpuscular hypothesis is true, or more seriously, for believing that it is wholly unable to explain the phenomena it purports to explain and therefore cannot be true.
First, he refers to the corpuscular hypothesis as a hypothesis, and one that falls well short of providing us with scientific knowledge. Further, he remarks that it is not his aim to adjudicate among competing hypotheses.
I have here instanced in the corpuscularian Hypothesis, as that which is thought to go farthest in an intelligible Explication of the Qualities of Bodies; and I fear the Weakness of humane Understanding is scarce able to substitute another, which will afford us a fuller and clearer discovery of the necessary Connexion, and Co-existence , of the Powers, which are to be observed united in several sorts of them. This at least is certain, that which ever Hypothesis be clearest and truest, (for that it is not my business to determine,) our Knowledge concerning corporeal Substances, will be very little advanced by any of them, till we are made see, what Qualities and Powers of Bodies have a necessary Connexion or Repugnancy one with another; which in the present State of Philosophy, I think, we know but to a very small degree (E IV.iii.16, pp. 547–548).
The hypothetical status of all physical theories is underscored also in Some Thoughts Concerning Education : “The systems of natural philosophy …are to be read, more to know the hypotheses …than with hopes to gain thereby a comprehensive, scientifical, and satisfactory knowledge of the works of nature” (Locke, quoted in Rogers 1982, p. 230). Still, while all physical theories are ultimately hypotheses, it is useful to bear in mind Peter Anstey’s remarks about this one’s genesis. The hypothesis originated among theorists championing an experimental method over a purely speculative one, and while they did not exclude speculation entirely, they circumscribed its role, engaging in it only after observation and experiment had already given the theory a solid foundation. Furthermore, the corpuscular hypothesis had credibility insofar as its theorists avoided the question about matter’s infinite divisibility and thereby adhered to their prohibition against questions they thought could never be answered experimentally (see Anstey, 2011, pp. 4–5).
Second, if Locke indeed identifies material bodies’ real essences with the primary qualities of their constituent corpuscles, that view of real essences combined with his pessimism about ever discovering them implies pessimism about the corpuscular hypothesis. Specifically, it implies pessimism about the claims that bodies are made of corpuscles and that those bodies’ observable qualities are reducible to the corpuscles’ qualities. In the same passages where Locke seems to support or assume the corpuscular hypothesis’s central tenets—that observable bodies are made up of corpuscles and that those corpuscles have a restricted set of inherent properties—he simultaneously appears very skeptical about the possibility of reducing observable properties such as color and taste to that restricted set of primary properties.
Third, Locke arguably believes that the corpuscular hypothesis’s limitations are so serious that they amount to fatal flaws, an interpretation that Wilson (1979) was perhaps the first to defend. Although Wilson develops her line of argument mainly in connection with difficulties Locke raises about the corpuscular hypothesis’s purported ability to explain sensation and more generally, the relation between thought and matter, [ 17 ] some other phenomena are troublesome as well. Locke appears to consider such phenomena so obscure that we can attempt to understand them only by attributing them to God’s direct action.
The coherence and continuity of the parts of Matter; the production of Sensation in us of Colours and Sounds, etc. by impulse and motion; nay, the original Rules and Communication of Motion being such, wherein we can discover no natural connexion with any Ideas we have, we cannot but ascribe them to the arbitrary Will and good Pleasure of the Wise Architect (E IV.iii.29, pp. 559–560).
Elsewhere, Locke will use the term ‘superaddition’ to refer to God’s role. For the moment, superadded properties may be neutrally described as those that God confers by fiat. Section 3.3., which reviews some of the main positions in the debate about Locke’s stance toward the corpuscular hypothesis, will also address the various interpretations of superaddition. [ 18 ] First, however, we will examine the problematic phenomena.
This section examines the four phenomena that Locke seems to consider too obscure for the corpuscular hypothesis to illuminate. The above-quoted passage mentioned three of these phenomena— the production of sensation, the communication of motion, and cohesion. [ 19 ] Locke discusses the fourth, gravity, only outside the Essay .
3.2.1 Sensation
As we saw in passages discussed earlier, in connection with scientia’s impossibility, Locke finds the production of sensation to be utterly obscure. One side of the difficulty is the nature of the mind. While in all probability, it is immaterial, Locke allows the possibility that God superadded the power of thought directly to matter. The other side of the difficulty concerns the nature of secondary qualities as powers to produce sensations. The appeal of the corpuscular hypothesis lay largely in its reductive promise. Reduction was expected particularly for secondary qualities, such as colors and sounds, but was also anticipated for ideas of macro-level primary qualities, including visual sensations of shapes and sizes, and tertiary qualities. All would be reduced to the primary qualities of bodies’ component corpuscles as they interact with one another and our perceptual systems.
One part of the corpuscular hypothesis’s purported explanation is conceivable, namely, the interactions among the primary qualities of bodies, which are supposed to be part of the causal basis of our sensations:
That the size, figure, and motion of one Body should cause a change in the size, figure, and motion of another Body, is not beyond our Conception; the separation of the Parts of one Body, upon the intrusion of another; and the change from rest to motion, upon impulse; these, and the like, seem to us to have some connexion one with another (E IV.iii.13, p. 545).
Indeed, we are capable of discerning necessary connections in two instances, as noted earlier. (One case involves only primary qualities— “Figure necessarily presupposes Extension” (E IV.iii.14, p. 546)—while the other involves tertiary and primary qualities—“receiving or communicating Motion by impulse, supposes Solidity” (E IV.iii.14, p. 546).) If we knew more about the primary qualities of bodies, we might multiply such instances: “And if we knew these primary Qualities of Bodies…we might be able to know a great deal more of these Operations of them one upon another”. That is, if we knew real essences, we could derive more necessary connections, to know the causal relation between opium and sleep, for instance, and as certainly as we now know that impulse requires solidity.
Still, knowing real essences would not give us any genuine knowledge of how sensations are produced by primary qualities. While corpuscular theorists such as Galileo ( The Assayer ) sketched a reductive account of our sensations of taste in terms of particles striking our tongues, Locke suggests that any attempt to discover the process’s details will be foiled. For as far as we can imagine, a body that strikes other bodies can produce “nothing but Motion” [ 20 ] (E IV.iii.6, pp. 540–541), and motion may itself be hopelessly obscure, as indicated below. The roles of shape and size are equally obscure; we cannot imagine how they could help produce sensations.
We are so far from knowing what figure, size, or motion of parts produce a yellow Colour, a sweet Taste, or a sharp Sound, that we can by no means conceive how any size, figure, or motion of any Particles, can possibly produce in us the Idea of any Colour, Taste , or Sound whatsoever; there is no conceivable connexion betwixt the one and the other (E IV.iii.13, p. 545).
Although Locke mentions only secondary qualities here, his point presumably applies to all sensations, including our sensations of macro-level primary qualities, such as the shape and size of a snowball or lump of gold. For again, genuine knowledge is knowledge of necessary connections, with the conceptual relations in geometry being the model, and it does not seem possible to discover such connections between any sensation and the sizes, shapes, and textures that are alleged to cause them. Macro-level primary qualities to micro-level ones and accordingly resemble them. [ 21 ] but the idea of a quality is nonetheless very different than the quality itself.
Locke finds that our only way of understanding the production of sensation is to attribute the process to God. If we try to understand how motion could produce a color, sound, or taste, “we are fain to quit our Reason, go beyond our Ideas , and attribute it wholly to the good pleasure of our Maker” (E IV.iii.6, p. 540–541; see also IV.iii.28, p. 559). Locke was certainly not alone in grappling with this problem. Commenting upon Descartes’s account, Walter Charleton (1654, p. 197) had observed earlier that even a detailed knowledge of light behavior would still leave us with the “superlative difficulty” of understanding why a certain reflection or refraction should be “transformed into a Vermillion rather than a Blew,” and further details about our sensory apparatus do not reveal “any Analogy betwixt the Retina Tunica … and any one Colour”. Locke was also not alone in resorting to a divine cause; in De gravitatione ’s creation account of bodies, for example, Newton suggested a divine basis for bodies’ abilities to stimulate perceptions in minds.
3.2.2 Gravity
Newton’s Principia implied the possibility of unmediated action at a distance, and with its publication, gravity became the most nettlesome phenomenon for the orthodox version of the corpuscular hypothesis due to its proviso of contact action. Locke was initially sympathetic to the proviso, writing in the first three editions of his Essay , “How bodies operate one upon another…is manifestly by impulse and nothing else. It being impossible to conceive that body should operate on what it does not touch ” (E II.viii.11, editions 1–3). Yet for the fourth edition, he replaced that claim about how bodies do operate with one about how we can conceive of them operating: “How Bodies produce ideas in us is manifestly by impulse , [this being] the only way which we can conceive Bodies [to] operate” (E II.viii.11, edition 4). He also omitted a clause denying unmediated action at a distance, which had appeared in II.viii.12 of previous editions. [ 22 ] These subtle emendations reflect a dramatic shift, one expressed directly in his correspondence with Stillingfleet.
The gravitation of matter towards matter, by ways inconceivable to me, is not only a demonstration that God can, if he pleases, put into bodies powers and ways of operation, above what can be derived from our idea of body, or can be explained by what we know of matter, but also an unquestionable and every where visible instance, that he has done so ( Second Reply to the Bishop of Worcester , 1699, The Works of John Locke , Vol. IV, p. 467).
The phenomenon of gravity—as explained by “Mr. Newton’s incomparable book” ( ibid. )—apparently led Locke to abandon the contact action proviso and to attribute to matter the power of acting distantly, even though he considered the process by which such interactions could occur so obscure that it drove him to invoke superaddition. This is the prevailing interpretation of Locke (and was assumed by Leibniz, who targeted Locke for it in Against Barbaric Physics ), though not all commentators agree, as indicated in a subsequent section.
3.2.3 Motion
Locke takes the notion of impulse, in which bodies communicate motion to one another by surface impact, to be, along with extension and cohesion, fundamental to our concept of body. [ 23 ] Indeed, regardless of how motion may actually be communicated, impulse is the only means by which we can conceive of its being communicated, a view of our conceptual abilities that Locke maintains, as we saw, despite his changing thoughts about gravity. Impulse is also fundamental to the corpuscular hypothesis’s explanation of phenomena, being either the exclusive means of interaction among bodies, as adherents of the contact action proviso hold, or the means of at least many interactions. Yet how exactly does a moving body communicate motion to a resting one simply by impacting it? When we attempt to discover the precise nature of the process, Locke suggests, we find that it is just as mysterious as the process by which the mind moves the body.
Another Idea we have of Body, is the power of communication of Motion by impulse ; and of our Souls, the power of exciting of Motion by Thought …. But if here again we enquire how this is done, we are equally in the dark . For in the communication of Motion by impulse, wherein as much Motion is lost to one Body, as is got to the other, which is the ordinariest case, we can have no other conception, but of the passing of Motion out of one Body into another; which, I think, is as obscure and unconceivable, as how our Minds move or stop our Bodies by Thought….The increase of Motion by impulse, which is observed or believed sometimes to happen, is yet harder to be understood. We have by daily experience clear evidence of Motion produced both by impulse, and by thought; but the manner how, hardly comes within our comprehension; we are equally at a loss in both (E II.xxiii.28, p. 311).
Since the corpuscular hypothesis holds impulse to be the primary if not the sole means by which bodies causally interact, then any phenomenon that the corpuscular hypothesis purports to explain by impulse will remain obscure if impulse itself remains obscure. All of the hypothesis’s reductions of observable primary, secondary, and tertiary qualities, would inherit impulse’s obscurity; thus Locke appears to be suggesting here that the corpuscular hypothesis cannot fulfill its promise of explaining and reducing those properties and powers.
3.2.4 Cohesion
Since the claim that observable bodies are made up of particles is central to the corpuscular hypothesis, an immediate question for its proponents asks how the particles cohere into compound bodies. Plenists have some resources for answering that question, though those resources may introduce worse difficulties. Descartes, for instance, though he speaks in terms of particles, understands an individual body as an area of extension moving as one with respect to surrounding areas. It is not possible for any particle to move away into empty space, there being no such thing as empty space once matter is identified with extension; with every bit of matter pressed from all sides by other matter, there is no problem about cohesion per se, though there is certainly a problem about individuating bodies from one another. In the same vein, Malebranche can invoke the pressure of the air to explain the coherence of bodies, and then invoke the pressure of an aether to explain the coherence of air particles. Locke objects that this explanation fails because it leaves us with the question of what causes the particles of the aether to cohere (E II.xxiii.23, p, 308). The objection reveals Locke’s atomist sympathies, drawing its power from the presumption that there is such a thing as empty space into which the aether particles could move. The problem for atomist versions of the corpuscular hypothesis is that the restricted set of properties that they allow the particles —size, shape, and motion—provides no obvious resources for explaining how the particles cohere with one another to form compound bodies. In various forms, the problem about cohesion has dogged atomists since ancient times.
The problem arises in two forms, which, to borrow James Hill’s terminology (Hill 2004), may be called the limited and the foundational problems. The limited problem, arising for those who take corpuscles to be genuine atoms, that is, to be indivisible, is the problem of explaining how those indivisible corpuscles cohere with one another. This is the problem one finds in Newton’s writings. Although Rule 3 of the Principia allows the possibility that the least parts of matter could turn out to be divisible, his atomist sympathies are evident throughout his writings. He speculates in Query 31 that in all probability, bodies are made up of hard particles that only God could divide, and in the body of the Opticks (Book II, Part III, Proposition VII) he suggests that more powerful microscopes might permit us to see the larger particles. In answer to the problem of how those naturally indivisible particles cohere, he rejects the ancient solution of hooked particles as begging the question, proposing instead some short-range forces modeled on the gravitational force (Query 31). Newton’s speculations about such forces are driven by an absence of any resolution to the problem about cohesion within the corpuscular theory itself.
The foundational problem pushes the question about cohesion into the corpuscles themselves. The problem was raised by Joseph Glanvill: “If it be pretended…that the parts of solid bodies are held together by hooks , and angulous involutions; I say, this comes not home: For the coherence of the parts of these hooks …will be of as difficult a conception, as the former” (Glanvill, The Vanity of Dogmatizing , p. 18, quoted in Hill 2004, p. 616). Without any grounds for asserting that the divisibility of matter bottoms out in indivisible corpuscles, then, the question arises of how the parts of a corpuscle could cohere, how the parts of those parts could cohere, and so on, ending in the question of how extended bodies are possible at all.
Whatever his ultimate view of the corpuscular hypothesis, Locke inevitably faces the atomist’s problem about cohesion, accepting as he does void space (see II.xiii.11, 12–14, 21–23), and holding that our ideas of body depend fundamentally upon cohesion. One of the ideas “proper and peculiar” to body, he writes, is “ the cohesion of solid , and consequently separable parts ” (E II.xxiii.17, p. 306), and the extension of body, as opposed to the extension of space, is “nothing, but the cohesion or continuity of solid, separable, moveable Parts” (E II.iv.5, p. 126). Yet we have no understanding of cohesion, and so our idea of body does not rest on any genuine understanding of it. In trying to understand how bodies are extended, we are as much in the dark as when we try to understand how the soul thinks.
’Tis as easie for him to have a clear Idea , how the Soul thinks, as how Body is extended . For since Body is no farther, nor otherwise extended, than by the union and cohesion of its solid parts, we shall very ill comprehend the extension of Body, without understanding wherein consists the union and cohesion of its parts; which seems to me as incomprehensible, as the manner of Thinking, and how it is performed (E II.xxiii.24, p. 309).
One scholarly debate about cohesion concerns the question of whether Locke acknowledged only the limited problem, as one would expect from commentators who read him as accepting atomism (e.g. Mandelbaum 1964, p. 1), or whether he looked further, to the more serious, foundational problem, as Hill argues (2004). A related controversy, to be discussed in the next section, concerns the question of whether Locke concludes that the corpuscular hypothesis simply cannot resolve the problem (e.g., Hill 2004; Downing 2007, p. 408) or instead remains agnostic on the issue (e.g., McCann in Chappell 1998, p. 244).
The four problematic phenomena winnow away the components of the corpuscular hypothesis, as defined at the outset of this article. The problem about sensation threatens the corpuscular hypothesis’s promise of reducing secondary, tertiary, and macro-level primary qualities to microlevel primary qualities. Newton’s results about gravitational phenomena cast grave doubts upon the contact action proviso, and according to some commentators, those results led Locke to abandon the belief that impulse is the only means of causal interaction. A corpuscular theorist might hope to preserve some part of the theory by insisting that impulse is still the means by which most other causal interactions are effected; but this runs up against the problem about impulse, in that the process by which motion is communicated seems utterly obscure. Finally, even the core claim that observable bodies are composed of tiny corpuscles is threatened by the problem of cohesion. This last problem threatens to be the most serious of four phenomena, for as James Hill has pointed out (2004, p. 628), the problems about gravity, sensation, and motion arise subsequent to our having conceived of body, whereas the problem about cohesion may thwart our very ability to conceive clearly of body.
This section considers some main responses to the tension between Locke’s seeming acceptance of the corpuscular hypothesis, most notable in his apparent identification of a material substance’s real essence with the size, shape, and texture of its insensible parts, and his pessimism about the hypothesis’s explanatory power, most notable in his remarks about the four phenomena discussed above.
One approach to the tension is to understand it as a genuine inconsistency. Margaret Wilson has defended such an interpretation, though in the 1979 paper that launched the debate, her intent is to show how acutely Locke understood the explanatory limitations of “Boylean mechanism”. Specifically, Wilson argues, the inconsistency reveals Locke’s recognition “that some presumed properties of matter cannot be conceived as ‘natural’ consequences of Boylean primary qualities” (Wilson 1979, p. 197). Thus, our ignorance about bodies has more profound causes than our ignorance about the primary qualities of a body’s constituent corpuscles. In accordance with her view that Locke’s agnostic tendencies (just like his dogmatic ones) are genuine, Wilson interprets Locke’s concept of superaddition robustly, as a sort of divine action that goes beyond the corpuscular hypothesis. According to this “non-essentialist” or “divine annexation” reading, Locke understands superadded properties as properties that God has annexed to matter by fiat, and that bear no intrinsic connection to matter’s real essence. This reading implies a distinction in etiology for superadded qualities. Whereas the other qualities of matter are either given initially, as those qualities constituting the real essence, or else flow from the real essence, superadded qualities are added on independently of the real essence, such that the substance would have been complete without them. This view implies that while superadded properties are consistently present, they are inexplicable by physical theory and, accordingly, are evidence of divine action. [ 24 ]
Some other interpretations absolve Locke of inconsistency, either by emphasizing his so-called dogmatic side while downplaying or reinterpreting his agnostic tendencies, or emphasizing his agnosticism while downplaying his dogmatism. One line of interpretation, then, reads Locke as in some manner accepting the corpuscular hypothesis (Mandelbaum 1964, chapter 1; Osler 1970, p. 12; Ayers 1975; McCann 1994, §1 and p. 85; McCann 2002, pp. 354–355). According to weaker versions of this reading, Locke’s project is the naturalistic one of pursuing the philosophical implications of the best available scientific theory, and developing an epistemological basis for it. McCann, for example, reads Locke as defending the atomist version of the corpuscular philosophy over its Cartesian competitor by providing an epistemology for it. While Descartes had provided an epistemology for his plenist version, there was nothing comparable for the atomist version associated with Gassendi and Boyle until Locke supplied it (McCann 2002, pp. 354–355). According to Ayers’ stronger interpretation, Locke accepts “pure mechanism,” that is, the orthodox version of the corpuscular hypothesis, which includes the contact action proviso. According to this view, all of matter’s qualities flow from its real essence (Ayers 1981).
Since this line of interpretation seeks to downplay Locke’s agnostic tendencies, one challenge is to account for Locke’s pessimism about the possibility of our knowing real essences. Mandelbaum meets the challenge by confining Locke’s pessimism to the real essences of particular material substances; we are able to know “the general properties possessed by all material substances,” and are ignorant only of the “particular sizes, shapes, number, or motions of the particles which go to make up any specific object” (Mandelbaum 1964, p. 54). A related challenge is to account for Locke’s appeals to superaddition, since prima facie , Locke’s reason for invoking God is that he thinks the corpuscular hypothesis has no resources for explaining the four problematic phenomena. Ayers responds by rejecting Wilson’s divine annexation interpretation of superaddition in favor of a deflationary one. According to Ayers’ “divine architect” interpretation, Locke makes no distinction in etiology by calling a property superadded; he means only that God selected the property with particular care when first creating matter. To diffuse the effect of Locke’s remarks to Stillingfleet, in which Locke appears to embrace action at a distance, Ayers points to Locke’s late manuscript, “The Elements of Natural Philosophy,” interpreting certain passages as referring gravitational effects to an undetectable medium (Ayers 1981, pp. 212–214). This move has been challenged by Stuart. Claiming that the manuscript was probably written for the education of a child, Stuart denies that it could trump Locke’s remarks to Stillingfleet (see Stuart 1998, pp. 378–379).
Another way to absolve Locke of inconsistency is to emphasize his agnosticism or skepticism, while downplaying or reinterpreting passages that appear to commit him to the corpuscular hypothesis. Interpretations in this vein tend to emphasize Locke’s pessimism about our ability to know real essences, to discern necessary connections, and consequently, have scientia in natural philosophy. Commentators pursuing this line include Downing (1998, 2007), Jolley (2002), and Connolly (2015); the latter argues for an even greater epistemic humility, one extending to real essences, along with superaddition (mentioned briefly below).
The central challenge facing such interpretations is to account for the passages in which Locke speaks as though he accepts the corpuscular hypothesis, most notably those in which he appears to identify the real essences of material substances with the corpuscles’ primary qualities. Jolley (2002) accounts for Locke’s so-called dogmatic tendency in strategic terms. Locke’s Essay targets both Aristotelians and Cartesians, and though agnosticism, which targets the Cartesians, is ultimately the dominant tendency in his thought, Locke emphasizes the explanatory power of the corpuscular hypothesis whenever he has the Aristotelians in his sights. Downing (1998, 2007), meanwhile, interprets Locke’s Essay as developing metaphysical distinctions that constrain physical theory, and then downplays his dogmatic side by taking the corpuscular hypothesis to be truly only a hypothesis for him, and denying that real essence can be identified with primary qualities. Properly understood, Downing argues, the distinction between real and nominal essence is a metaphysical distinction. It is thus more fundamental than the distinction between primary and secondary qualities, which belongs to a particular physical theory, the corpuscular hypothesis. To be worth its salt, a physical theory must meet the metaphysical constraint provided by the real-nominal essence distinction. That is, the physical theory must provide some way of making sense of the notion that material bodies have an internal constitution that is inaccessible to us while producing qualities that are accessible. Locke explains his metaphysical distinction using one physical theory, the corpuscular hypothesis, as an illustration, and he often appears to accept or even defend that hypothesis. Still, this is only an appearance, an appearance due to a certain advantage that the corpuscular hypothesis has over other physical hypotheses: it is the theory best suited to our sensory capacities and understanding. Despite its unique status, Locke sees it as a mere hypothesis, one crippled by the explanatory limitations evidenced by the problematic phenomena discussed earlier. For Downing, then, Locke’s dogmatic tendencies disappear, leaving only his agnostic side.
Taking a different approach to the situation, Jacovides (2017) treats Locke’s descriptions of what we perceive, intuit, and are capable of conceiving as providing a case for testing some Kuhnian theses; as the normal science of his day, the corpuscularian hypothesis sets limits upon what Locke can conceive.
4. Locke and Newton
Locke and Newton probably first met in 1689 (though the exact date is not known; Westfall 1980, p. 488; Rogers 1982, p. 219), and their main works were written independently of one another; Locke’s Essay ,though published subsequently, was essentially complete by the time he read the Principia . A noticeable intellectual affinity may nevertheless be seen in those works, and the opportunity for mutual influence followed as they established a friendship, exchanging views on a wide variety of subjects, not least certain unorthodox theological convictions (see Westfall 1980, 490–91). The influence did not run in one direction alone; in a draft passage probably penned shortly after the Principia ’s second edition, for example, Newton adopts a Lockean tone as he denies that any ideas are innate. [ 25 ] As for Newton’s influence upon Locke, the best-known example concerns action at a distance, as noted below, though there are deeper questions about methodology.
A good deal of resemblance to Locke’s epistemological approach can be seen in Newton, who holds that, revelation apart, we must gather what knowledge we can from our perceptions, and anything like a real essence eludes us. In the early manuscript, De gravitatione , for instance, Newton denies knowing the “essential and metaphysical constitution” of matter (Newton, 2004, p. 27). He reiterates this position in later texts, including the 1713 General Scholium:
We certainly do not know what is the substance of any thing. We see only the shapes and colors of bodies, we hear only their sounds, we touch only their external surfaces….But there is no direct sense and there are no indirect reflected actions by which we know innermost substances ( Principia , 942).
Locke and Newton also share the problem of an evidentiary deficit, insofar as they subscribe to corpuscularianism. Thinkers who did subscribe tended to rely on transduction (also called transdiction)—an inductive inference that is empirical insofar as it relies upon observed cases, but which generalizes not only to unobserved cases but to those that are unobservable. [ 26 ] ) Newton’s Rule 3 licensed such inferences, from the Principia ’s second edition onward, for qualities of invariable intensity, i.e., extension, impenetrability, hardness, mobility, and the vis inertiae . The rule explicitly permits inferences to the realm of the unobservable: “Because the hardness of the whole arises from the hardness of the parts, we justly infer from this not only the hardness of the undivided particles of bodies that are accessible to our senses, but also of all other bodies” ( Principia , Book 3, p. 795 [ 27 ] ). As for Locke and the problem of transduction, how serious it is for him depends largely upon the status of the corpuscular hypothesis. If he takes an agnostic or skeptical stance toward it, then he owes no solution to the problem.
Although the endeavors of Locke and Newton are often considered complementary, there are questions about how Locke responded to the Principia , both in terms of its methodology and its epistemological implications. With regard to the former, how far did Locke go in absorbing or incorporating the methodology that Newton had forged? As a number of commentators have emphasized, the new science was itself in flux at this time, with natural history being gradually sidelined by Newton’s approach, an experimentalism that was theoretical and mathematical (see, e.g., Anstey 2011; Roux, 2013). Given that Locke had had Boyle as his mentor but was greatly impressed by Newton’s Principia , it is natural to ask whether a similar shift was occurring in Locke’s own thought. Although Locke was influenced by Newton, his allegiances to certain older ideas ran deep. De Pierris (2006) explains Locke’s failure to adopt the method of inductive proof in terms of his devotion to the ideal of demonstrative knowledge, as combined with a belief in hidden primary qualities.
With regard to its epistemological implications, what precisely did Locke take the Principia to have achieved? To pose the question in the starkest possible terms, might Newton’s Principia have tempted Locke to retreat from his belief that natural philosophy cannot be made a science (much as it led him to retreat from the contact action proviso)? Perhaps Locke categorized Newton’s epistemological achievement in natural philosophy as a contribution to sensitive knowledge alone. That is, perhaps he saw Newton’s “mighty Designs in advancing the Sciences” ( Essay , Epistle to the Reader, pp. 9–10) as confined to providing a firm basis for natural philosophy through his experimental method. But did he perhaps instead take Newton’s mathematical methods as offering the demonstrations needed to push natural philosophy into the domain of demonstrative knowledge and hence scientia?
Commentators interpreting Locke as seeing Newton’s contribution in terms of sensitive knowledge alone include Yolton (1969); Woolhouse (1994); and Downing (1997, see especially pp. 292–93). Winkler (2008), however, reads Locke’s correspondence with Stillingfleet and other writings from the 1690s as a defense of Newton’s mathematical physics, and one that draws from his mathematical demonstrations a greater optimism about the possibility of certain knowledge in natural philosophy. This move has been contested by Domski (2012), who argues that Locke advocated Newton’s mathematical methods only in connection with astronomy, whose objects are unavailable for experimentation; and that Locke maintained his emphasis upon natural historical methods for questions about terrestrial bodies. The debate over the extent to which Locke prioritized natural history over experimentalism directly concerns his stance on hypotheses (a controversy mapped by Priselac, 2017), while a related debate concerns the relationship between medicine and natural philosophy. Opposing Yost (1951) among others, Lu-Adler (2021) has recently argued that Locke did not assume that the scientific methodology most appropriate to medicine could be generalized to physics.
Locke’s discussions of space, body, mind, and God bear some striking similarities to Newton’s, raising questions about the extent of those similarities as well as points of influence. Newton presents his mature concept of space in the Principia , having formulated important aspects of it earlier in the manuscript De gravitatione . In both texts, space is distinct from body and is real, infinite, three-dimensional, and homogeneous. Whereas body has separable parts, Newton’s eternal, infinite space is immobile and indivisible, having merely distinguishable parts. Although space is neither substance nor attribute, it would be a mistake to suppose that it is nothing; as he emphasizes in De gravitatione (2004, p. 21, 22), by explaining space’s properties he has shown that it is something.
Unlike Newton, Locke initially accepted a relationist view of space; in journal entries of 1676–78 and the Early Draft of the Essay, space is nothing more than a relation between bodies that do not touch (see Gorham, 2020, p. 221). In the Essay , however, his discussion of space and its relation to body is strongly reminiscent of the Principia ’s absolutist conception. Locke confesses himself to be among those “who persuade themselves … that they can think on Space, without anything in it, that resists, or is protruded by Body” (E II.iii.5, p. 126). Declaring that explicating extension in terms of partes extra partes would be tautological (E II.iii.15, p. 173) and declining to answer the question of whether space is substance or accident (E II.iii.17, p. 174), Locke describes our idea of “pure Space” (E II.iii.5, p. 126) as an extension consisting of “the continuity of unsolid, inseparable, and immoveable Parts” ( ibid .; see also E II.xiii.13, 14, which further discuss space’s perpetual rest and the impossibility of separating its parts, either actually or mentally). Lacking solidity, space poses no resistance to a body’s motion (E II.xiii.12, p. 172). Again recalling Newton, who takes space to be a consequence of God’s existence (2004, p. 21), Locke invokes God when defending his conception of space: “Those who assert the impossibility of Space existing without Matter, must not only make Body infinite, but must also deny a power in God to annihilate any part of Matter” (E II.xiii.21, p. 176).
Analyzing remarks such as the above, most commentators have concluded that by the time of the published Essay , Locke had fully abandoned the relationist view (see Gibson, 1960; Di Biase, 2016; Gorham and Slowik, 2014; Gorham, 2020). However, Locke tends to describe our ideas rather than things themselves, and the gap between the two has led a few commentators to conclude that Locke retained his earlier relationism or, at least, some inclination toward it (e.g., Thomas, 2016; see also Rogers, 1978).
Locke’s concept of body also overlaps considerably with Newton’s. In developing a concept of body, Locke notoriously finds the related, general problem about a substratum or substance vexing, [ 28 ] whereas Newton dispenses with the problem more easily. In De gravitatione , Newton eliminates the unintelligible notion of prime matter by associating perceived properties with determined quantities of extension. [ 29 ] Both thinkers attack Descartes’ identification of matter with extension, instead sympathizing strongly with the notion of atoms in a void. Further, Newton’s list in Rule 3 of body’s universal qualities includes not only extension, hardness, impenetrability, and mobility, but also the vis inertiae —the inherent force or power of resisting ( Principia , Definition 3), which some commentators take Newton to identify with mass. [ 30 ] Locke’s concept, however, emphasizes extension, mobility, and solidity, without mentioning mass. Still, since Locke explicates solidity in terms of impenetrability, which arises from resistance (E II.iv.1), there may be grounds for holding that his concept does after all include mass, a position defended by Woolhouse (2005). [ 31 ] At the least, Locke’s discussion of action by impulse presupposes mass, as Stein has noted. Nevertheless, presupposing the concept may not amount to including it in the concept of body, since as Stein points out, mass for Locke “cannot be construed to correspond to a simple idea, but can only be understood as a power ‘mediately perceivable’” (Stein 1990, p. 36).
The question about gravity’s relation to body is also a persistent source of controversy for both thinkers. [ 32 ] One point of clarity and consensus is that neither Locke nor Newton considers the power of gravitational attraction to be essential to matter. Newton consistently denies that it is essential (for instance in his explanatory remarks following Rule 3 of the Principia ) and Locke refers to it only as power superadded or endowed by God. Locke’s concept of superaddition is controversial, as noted earlier, but he does appear to embrace action at a distance in his letter to Stillingfleet. Newton’s writings contain no such dramatic pronouncements. So, while a few commentators interpret Newton as accepting action at a distance, grounded either in a superadded property (Henry 1994) or in a relational quality of matter (Schliesser 2011), his more favorable remarks are at best indirect. Most commentators interpret him as at least having very grave misgivings about distant action, so perhaps Locke was wise to credit his change of heart about gravity to “Mr. Newton’s incomparable book,” rather than to Newton himself.
With respect to mind, Locke and Newton are both circumspect about its nature and yet may be considered substance dualists. Although Newton indicates in De gravitatione that he does not pretend to know the substantial foundation of minds, he consistently presents minds as immaterial, lacking certain characteristics of body, namely, hardness, impenetrability and resistance. Similarly, while Locke explicitly allows the possibility of thinking matter in his Essay and discusses it at length with Stillingfleet, he emphasizes that in all probability, the soul is immaterial (E IV.iii.6, pp. 540–541). Does Locke share Newton’s view that even immaterial spirits are spatially extended, such that a mind can co-occupy place with a body? A Cartesian interpretation does not seem impossible given Locke’s remarks about the possibility of thinking matter, which contrast matter against an immaterial soul that is unextended. [ 33 ] Still, at several passages in the Essay , Locke seems to agree with Newton. At E II xxvii.2 (p. 329), he locates minds spatially: “Finite spirits having had each its determinate time and place of beginning to exist, the relation to that time and place will always determine to each of them its identity, as long as it exists”.
Finally, Locke seems to share Newton’s belief in God’s substantial ubiquity and its implications. As the above-quoted passage’s discussion of finite spirits continues, he remarks more generally on the possibility of co-presence: “These three sorts of substances, as we term them, do not exclude one another out of the same place” ( ibid .). His later remarks about the “infinite Space … possessed by God’s infinite Omnipresence” (E II.xvii.20, p. 222) are similar, but by framing them in terms of third parties’ ideas, Locke leaves more room there for interpretive controversy.
Primary Literature
- Boyle, R., 1666, The Origine of Formes and Qualities (According to the Corpuscular Philosophy) , Oxford: H. Hall.
- Charleton, W., 1654, Physioliogia Epicuro-Gassendo-Charltoniana, or a Fabrick of Science Natural upon a Hypothesis of Atoms. Printed by Tho: Newcomb, for Thomas Heath.
- Galileo, 1623, “The Assayer,” in M.R. Matthews (ed.), The Scientific Background to Modern Philosophy: Selected Readings , Indianapolis: Hackett, 1989.
- Locke, J., 1975 [1700], An Essay Concerning Human Understanding , P. H. Nidditch (ed.), based on the fourth edition, New York: Oxford University Press.
- –––, 1824, The Works of John Locke , in nine volumes, 12 th edition, London: C. and J. Rivington.
- Newton, I., 1999 [1726], The Principia: Mathematical Principles of Natural Philosophy , trans. I. Bernard Cohen and Anne Whitman, Berkeley: University of California Press.
- Newton, I., 2004, Newton: Philosophical Writings , ed. Andrew Janiak, Cambridge: Cambridge University Press, 2004.
Secondary Literature
- Anstey, Peter, 2011, John Locke and Natural Philosophy , Oxford: Oxford University Press.
- Atherton, M., 1991, “Corpuscles, Mechanism, and Essentialism in Berkeley and Locke,” Journal of the History of Philosophy , 29 (1): 47–67.
- Ayers, M.R., 1975, “The Ideas of Power and Substance in Locke’s Philosophy,” Philosophical Quarterly , 25 (98): 1–27.
- –––, 1981, “Mechanism, Superaddition, and the Proof of God’s Existence in Locke’s Essay,” The Philosophical Review , 90 (2): 210–251.
- Clarke, D.M., 1992, “Descartes’ philosophy of science,” in J. Cottingham (ed.), The Cambridge Companion to Descartes , Cambridge: Cambridge University Press, 258–285.
- Cohen, I.B., 2002, “Newton’s concepts of force and mass, with notes on the Laws of Motion,” in I. Bernard Cohen and George E. Smith (eds.), The Cambridge Companion to Newton , Cambridge: Cambridge University Press, 2002, pp. 57–84.
- Connolly, P.J., 2015, “Lockean Superaddition and Lockean Humility,” Studies in History and Philosophy of Science (Part A), 51: 53–61.
- Curley, E.M., 1972, “Locke, Boyle, and the Distinction between Primary and Secondary Qualities,” The Philosophical Review , 81 (4): 438–464.
- De Pierris, G., 2006, “Hume and Locke on Scientific Methodology: The Newtonian Legacy,” Hume Studies , 32(2): 277–330.
- Dear, P., 1995, Discipline and Experience: The Mathematical Way in the Scientific Revolution , Chicago: University of Chicago Press.
- Di Biase, G., 2016, “ Physica in John Locke’s Adversaria and Classification of the Branches of Knowledge,” Locke Studies , 16: 69–165. doi:10.5206/ls.2016.654
- Domski, M., 2012, “Locke’s Qualified Embrace of Newton’s Principia,” in Interpreting Newton: Critical Essays , ed. A. Janiak, and E. Schliesser, Cambridge: Cambridge University Press.
- Downing, L., 1997, “Locke’s Newtonianism and Lockean Newtonianism,” Perspectives on Science , 5(3): 285–310.
- –––, 1998, “The Status of Mechanism in Locke’s Essay ,” The Philosophical Review , 107 (3): 381–414.
- –––, 2007, “Locke’s Ontology,” in L. Newman (ed.), The Cambridge Companion to Locke’s Essay , Cambridge: Cambridge University Press, pp. 352–380.
- Duncan, S., 2021, “Locke, God, and Materialism,” Oxford Studies in Early Modern Philosophy , 10: 101–131.
- Gibson, J., 1960, Locke’s Theory of Knowledge and its Historical Relations , Cambridge: Cambridge University Press.
- Gorham, G., 2011, “How Newton Solved the Mind-Body Problem,” History of Philosophy Quarterly , 28: 21–44.
- Gorham, G. and Slowik, E., 2014, “Locke and Newton on Space and Time and their Sensible Measures,” in Z. Biener and E. Schliesser (eds.), Newton and Empiricism , Oxford: Oxford University Press.
- Gorham, G., 2020, “Locke on Space, Time, and God,” Ergo: An Open Access Journal of Philosophy , 7(7), 07 November 2020. doi:10.3998/ergo.12405314.0007.007
- Henry, J., 1994, “‘Pray do not ascribe that notion to me’: God and Newton’s Gravity,” in J.E. Force and R. H. Popkin (eds.), The Books of Nature and Scripture: Recent Essays on Natural Philosophy, Theology and Biblical Criticism in the Netherlands of Spinoza’s Time and the British Isles of Newton’s Time , Dordrecht: Kluwer Academic Publishers, pp. 123–147.
- Hill, J., 2004, “Locke’s Account of Cohesion and its Philosophical Significance,” British Journal for the History of Philosophy , 12 (4): 611 – 630.
- Jacovides, Michael, 2017, Locke’s Image of the World . Oxford: Oxford University Press.
- Janiak, A., 2008, Newton as Philosopher , Cambridge: Cambridge University Press.
- Jardine, N., 1991, “Demonstration, Dialectic, and Rhetoric, in Galileo’s Dialogue ,” in D.R. Kelley and R.H.Popkin, Shapes of Knowledge from the Renaissance to the Enlightenment , Berlin: Springer, pp. 101–121.
- Jolley, N., 2002, Locke: His Philosophical Thought , Oxford: Oxford University Press.
- Jones, J.-E., 2023, “Locke on Real Essence”, The Stanford Encyclopedia of Philosophy (Summer 2023 Edition), Edward N. Zalta & Uri Nodelman (eds.), URL = < https://plato.stanford.edu/archives/sum2023/entries/real-essence/ >.
- Kochiras, H., 2011. “Gravity’s Cause and Substance Counting: Contextualizing the Problems,” Studies in History and Philosophy of Science , 42(1): 167–184.
- –––, 2021, “Newton’s Matter Theory,” in E. Schliesser and C. Smeenk, The Oxford Handbook of Newton , 12 November 2021. doi:10.1093/oxfordhb/9780199930418.013.23
- Koyre, A., 1965, Newtonian Studies , London: Chapman & Hall.
- Langton, R., 2000, “Locke’s Relations and God’s Good Pleasure,” Proceedings of the Aristotelian Society , 100: 75–91.
- Lu-Adler, H., 2021, “Locke on Scientific Methodology,” in J. Gordon-Roth and S. Weinberg, The Lockean Mind , London: Routledge, pp. 277–289.
- Mandelbaum, M., 1964, Philosophy, Science, and Sense Perception , Baltimore: The Johns Hopkins Press.
- McCann, E., 1994: “Locke’s Philosophy of Body,” in V. Chapell (ed.) The Cambridge Companion to Locke , Cambridge: Cambridge University Press, pp. 56–88.
- –––, 2002, “John Locke,” in S. Nadler, A Companion to Early Modern Philosophy , Oxford: Blackwell Publishing.
- McGuire, J.E., 1970, “Atoms and the Analogy of Nature,” reprinted in J.E. McGuire, Tradition and Innovation: Newton’s Metaphysics of Nature (The University of Western Ontario Series in the Philosophy of Science), Dordrecht: Kluwer Academic, 1995.
- Nuovo, V., 2017, John Locke: The Philosopher as Christian Virtuoso , Oxford: Oxford University Press.
- Osler, M.J., 1998, “Mixing Metaphors: Science and religion or natural philosophy and theology in early modern Europe,” History of Science , 36: 91–113.
- Osler, M.J., 1970, “John Locke and the Changing Ideal of Scientific Knowledge,” Journal of the History of Ideas , 31 (1): 3–16.
- Ott, Walter, 2009, Causation and Laws of Nature in Early Modern Philosophy , Oxford: Oxford University Press.
- Palmieri, P., 1998 “Re-examining Galileo’s Theory of Tides,” Archiv. Hist. Exact Sci. 53 (1998) 223–375.
- –––, 2009, “A phenomenology of Galileo’s experiments with pendulums,” British Journal for the History of Science , 42(4): 479–513, December 2009.
- Park, K. and Daston, L., 2006, “Introduction: The Age of the New,” in K. Park and L. Daston (eds.), The Cambridge History of Science (Volume 3: Early Modern Science), Cambridge: Cambridge University Press.
- Priselac, Matthew, 2017, Locke’s Science of Knowledge , London: Routledge.
- Rogers, G.A.J., 1978, “Locke’s Essay and Newton’s Principia, ” Journal of the History of Ideas , 39(20): 217–232.
- –––, 1982, “The System of Locke and Newton,” in Z. Bechler (ed.), Contemporary Newtonian Research , Dordrecht: D. Reidel Publishing Co., pp. 215–238.
- Roux, S., 2013, “An Empire Divided: French Natural Philosophy (1670–1690),” in Garber and Roux (eds.), The Mechanization of Natural Philosophy , Dordrecht: Springer.
- Schliesser, E., 2011, “Without God: Newton’s Relational Theory of Attraction,” in D. Jalobeanu and P. Anstey (eds.), Vanishing Matter and the Laws of Motion: Descartes and Beyond , London: Routledge, pp. 80–100.
- Smith, R., 2009, “Aristotle’s Logic,” The Stanford Encyclopedia of Philosophy (Spring 2009 Edition), Edward N. Zalta (ed.), URL = < https://plato.stanford.edu/archives/spr2009/entries/aristotle-logic/ >.
- Stein, Howard, 2002 [2016], “Newton’s Metaphysics,” in I. Bernard Cohen and George E. Smith (eds.), The Cambridge Companion to Newton , Cambridge: Cambridge University Press, pp. 256–307; 2nd edition, Rob Iliffe and George E. Smith (eds.), Cambridge: Cambridge University Press, 2016, pp. 321–381.
- –––, 1993, “On Philosophy and Natural Philosophy,” Midwest Studies in Philosophy , 18 (1): 177–201.
- –––, 1990, “Locke, the Great Huygenius, and the Incomparable Mr. Newton,” in P. Bricker and R. I. G. Hughes (eds.), Philosophical perspectives on Newtonian science , Cambridge, MA: MIT Press, pp. 17–48.
- Stuart, M., 1998, “Locke on Superaddition and Mechanism,” British Journal for the History of Philosophy , 6 (3): 351–379.
- Thomas, E., 2016, “On the ‘Evolution’ of Locke’s Space and Time Metaphysics,” History of Philosophy Quarterly , 33(4): 305–325.
- Van Dyck, M., 2005, “The Paradox of Conceptual Novelty and Galileo’s Use of Experiments,” Philosophy of Science , (72)5: 864–875.
- Westfall, R. S., 1980, Never at Rest: A Biography of Isaac Newton , New York: Cambridge University Press.
- Wilson, M., 1991, “Superadded Properties: The Limits of Mechanism in Locke,” reprinted in Wilson, Ideas and Mechanism: Essays on Early Modern Philosophy , Princeton: Princeton University Press, pp. 196–214.
- –––, 1999, Ideas and Mechanism , Princeton: Princeton University Press.
- Wisan, W.L., 1978, “Galileo’s scientific method: a reexamination,” in R.E. Butts and J.C. Pitt (eds.), New Perspectives on Galileo , Dordrecht: D. Reidel, pp. 1–58.
- Winkler, K. P., 2008, “Locke’s Defense of Mathematical Physics,” Paul Hoffman, David Owen, and Gideon Yaffe (eds.), Contemporary Perspectives on Early Modern Science: Essays in Honor of Vere Chappell . Toronto: Broadview Press, 231–252.
- Woolhouse, R.S., 1971, Locke’s Philosophy of Science and Knowledge , Oxford: Blackwell.
- –––, 1994, “Locke’s Theory of Knowledge,” in Vere Chappell (ed.), The Cambridge Companion to Locke , New York: Cambridge University Press, 146–171.
- –––, 2005, “Locke and the Nature of Matter,” in C. Mercer and E. O’Neill (eds.), Early Modern Philosophy: Mind, Matter, and Metaphysics , Oxford: Oxford University Press, pp. 142–161.
- Yolton, J., 1969, “The Science of Nature,” in John W. Yolton (ed.), John Locke: Problems and Perspectives , Cambridge: Cambridge University Press, 183–193.
- Yost, R.M. 1951, “Locke’s Rejection of Hypotheses about Sub-Microscopic Events,” Journal of the History of Ideas 12 (1): pp. 111–130.
How to cite this entry . Preview the PDF version of this entry at the Friends of the SEP Society . Look up topics and thinkers related to this entry at the Internet Philosophy Ontology Project (InPhO). Enhanced bibliography for this entry at PhilPapers , with links to its database.
- Aristotle, Posterior Analytics , trans. G.R.G. Mure.
- Early Modern Texts .
- The Works of John Locke in Nine Volumes , The Online Library of Liberty.
- John Locke Resources , maintained by John C. Attig, Pennsylvania State University.
Boyle, Robert | demonstration: medieval theories of | Descartes, René | knowledge: analysis of | Locke, John | Newton, Isaac | Newton, Isaac: philosophy | primary and secondary qualities in early modern philosophy | rationalism vs. empiricism | substance
Copyright © 2023 by Hylarie Kochiras < kochiras @ gmail . com >
- Accessibility
Support SEP
Mirror sites.
View this site from another server:
- Info about mirror sites
The Stanford Encyclopedia of Philosophy is copyright © 2023 by The Metaphysics Research Lab , Department of Philosophy, Stanford University
Library of Congress Catalog Data: ISSN 1095-5054

What do the lock and key hypothesis state?
The lock and key hypothesis: the theory was postulated by emil fisher in 1898. according to the hypothesis, like a lock can be open by its key only, a substance possessing specific composition only can combine with the specific active site found in the specific enzyme’s surface. the molecules on which the activities of the enzymes take place are called substrates. the substrates possessing accurate geometric configuration can fit within the enzyme’s active site. the enzyme’s active site is highly specific to the surface of the substrate. the substrate and the enzyme fit like a lock and key, providing a lock and key enzyme action model. some of the molecules, which compete with the substrate can either cease or slow down the reaction. these substances are called competitive inhibitors..

What Represents Lock And Key In The Lock And Key Hypothesis?
Key and lock hypothesis of enzyme action was given by

Official websites use .gov
Secure .gov websites use HTTPS
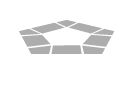
Service Chiefs Discuss Role of Partnerships in Meeting Defense Demands
Partnerships with allies and across the services are key to maintaining the United States' edge amid an increasingly challenging national security backdrop, the military's top officers said.
That message echoed as a central theme as the Joint Chiefs of the six armed forces branches came together yesterday for a panel discussion on U.S. defense priorities and military readiness hosted by the Council on Foreign Relations in Washington.
The leaders described a rapidly changing security landscape characterized by a range of challenges throughout the Indo-Pacific, Middle East and Europe.
Marine Corps Commandant Gen. Eric M. Smith said these challenges are becoming progressively linked as China, the United States pacing challenge, and Russia, which defense officials have identified as an "acute threat," attempt to challenge international rules-based order.
He said the U.S. military must stand ready to deter and, if necessary, defeat would-be aggressors on multiple, simultaneous fronts.
"Russia is an opportunistic aggressor," Smith said. "And so, if war breaks out with China, you can be sure that Russia will follow. And if war breaks out with Russia, you can be sure that China will follow.
"They're both opportunistic feeders and opportunistic aggressors," he said. "And they'll look for the seams in our armor — the chinks in armor — and they'll work to exploit those."
Smith said the Marine Corps accounts for these overlapping threats in shaping force for the future, while posturing primarily to counter the pacing challenge posed by China.
"For us, the force design is designed against the peer competitor of China," he said. "They are the pacing threat by the National Defense Strategy , so [the] force design is aimed at deterring China, and we still believe that that will include all the lesser included offenses such as Russia and [North Korea]."
He said his service's focus on modernized weapon systems and the ability to fight from longer ranges is useful for deterring and defeating aggression in any theater.
Air Force Chief of Staff Gen. David W. Allvin echoed Smith in highlighting the strategic deterrence challenge posed by Russia's longstanding nuclear capability coupled with China's rapid buildup of its nuclear arsenal.
He said maintaining a safe, effective and reliable nuclear triad remains critical for deterrence.
"I think what is going to be interesting for the nation, for the national security apparatus, is to really get a grip on what it really means to have strategic deterrence in a tri-polar type world," as China's nuclear capability approaches parity with the U.S.
He said deterring strategic threats in a tri-polar world is "fundamentally different" from deterrence in the past.
"That's a national conversation," he said. "But from the military side, we just have to make sure that our capabilities are suited to the task."
In recognizing the uptick in challenges, the service chiefs noted the key role partnerships will play in ensuring the U.S. maintains its military advantage.
Chief of Naval Operations Adm. Lisa Franchetti said the United States' partnerships with allies in the Indo-Pacific, in particular, are a key to managing competition with China.
Spotlight: Focus on Indo-Pacific
"I think the thing that really distinguishes us from any of our potential adversaries is that we do have allies and partners all over the world," she said. "And nowhere is that more important than in the Indo-Pacific."
"I think as we leverage our relationships that we already have, and we continue to build new ones in the Indo-Pacific, we will continue to develop the capabilities to deter China or any other adversary in the Indo-Pacific or around the world," Franchetti said.
U.S. Coast Guard Commandant Adm. Linda Fagan said partnerships were also key to deterring threats in the Arctic.
The Coast Guard, she said, has played a key role in bolstering these partnerships through exercises and capacity building among Arctic stakeholders.
"This is our nation's competitive advantage," Fagan said. "Not only do these nations welcome us, we're partners of choice."
Army Chief of Staff Gen. Randy A. George also highlighted the key role the NATO alliance will continue to play in Europe, and the importance of the bloc to continue to improve its collective capability.
"The battlefield is changing very rapidly," he said. "I think it's changing more in the last couple of years than I've ever seen it."
Spotlight: NATO
George said NATO's collective ability to respond quickly and effectively to the changing battlefield will be critical going forward.
In addition to underscoring the importance of partnerships among allies, Space Force Chief of Space Operations Gen. Chance Saltzman said the ability to work across the services is critical.
He said the service chiefs each espouse a commitment to partnership "because we know that's the only way to succeed."
He said that includes not only partnering with allies, but also partnering with industry and between the services.
"We know that each, individually, our forces, our services, our capabilities are inadequate to the global tasks that we've been presented," Saltzman said. "And if we can't work together — [...] technically, operationally, doctrinally — if we can't work together then we're in a world of hurt."
Spotlight: Value of Service Spotlight: Value of Service: https://www.defense.gov/Spotlights/Value-of-Service/
Subscribe to Defense.gov Products
Choose which Defense.gov products you want delivered to your inbox.
Related Stories
Defense.gov, helpful links.
- Live Events
- Today in DOD
- For the Media
- DOD Resources
- DOD Careers
- Help Center
- DOD / Military Websites
- Agency Financial Report
- Value of Service
- Taking Care of Our People
- FY 2025 Defense Budget
- National Defense Strategy
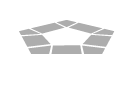
The Department of Defense provides the military forces needed to deter war and ensure our nation's security.
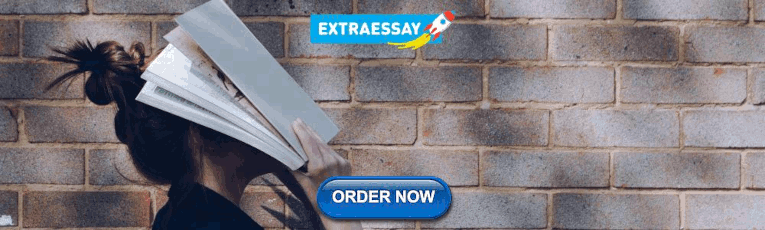
IMAGES
VIDEO
COMMENTS
Lock-and-key vs. Induced Fit Model. At present, two models attempt to explain enzyme-substrate specificity; one of which is the lock-and-key model, and the other is the Induced fit model.The lock and key model theory was first postulated by Emil Fischer in 1894.The lock-and-key enzyme action proposes the high specificity of enzymes.
Lock and Key Model. A German scientist, Emil Fischer postulated the lock and key model in 1894 to explain the enzyme's mode of action. Fischer's theory hypothesized that enzymes exhibit a high degree of specificity towards the substrate. This model assumes that the active site of the enzyme and the substrate fit perfectly into one another ...
1 Emil Fischer's Lock-and-Key Hypothesis after 100 Years- Friedrich Cramer Towards a Supracellular Chemistry 1 2 Molecular Recognition in Biology: Models for Analysis of Protein-Ligand Interactions 25 Doron Lancet, Amnon Horovitz and Ephraim Katchalski-Katzir 3 New Biocatalysts via Chemical Modification Ian M. Bell and Donald Hilvert 73
In 1894, Emil Fisher discovered that glycolytic enzymes are able to distinguish between sugar stereoisomers. Based upon that discovery, he formulated the lock-and-key hypothesis (Fischer 1894), which proposed that enzymes recognize their substrates just as a lock receives a key.That is, only in the case of exact geometric complementarity between the substrate (key) and enzyme (lock) is the ...
The Induced Fit Model Builds upon the Lock-and-Key Hypothesis. This lock-and-key model served the biochemical community well for over 50 years. However, while this model adequately explained how substrates that are too large to fit within the confines of the active site would fail to act as substrates, it did not explain how small substrates, for instance water, often acted as non-substrates ...
The theory behind the Lock and Key model involves the complementarity between the shapes of the enzyme and the substrate. Their complementary shapes make them fit perfectly into each other like a lock and a key. According to this theory, the enzyme and substrate shape do not influence each other because they are already in a predetermined ...
The current theory, known as the induced-fit model, says that enzymes can undergo a change in conformation when they bind substrate molecules, ... The lock-and-key model portrays an enzyme as conformationally rigid and able to bond only to substrates that exactly fit the active site. The induced fit model portrays the enzyme structure as more ...
The traditional Emil Fisher's 'lock-key' model uses analogy between enzyme (lock) and substrate (key) to describe the need for a matching shape of a substrate in order to fit to the active site of an enzyme [ 20 ]. The preference of an enzyme for given substrates is attributed to the quality of the match between enzyme active site and ...
The lock and key model is a hypothesis explaining how enzymes interact with substrates. It compares the specificity of enzyme-substrate interactions to a lock that only opens with the correct key. In this model, enzymes (locks) have specific active sites that perfectly fit their substrates (keys). Q. How does the lock and key model maintain ...
Other articles where key-lock hypothesis is discussed: chromatography: Retention mechanism: Very specific intermolecular interactions, "lock and key," are known in biochemistry. Examples include enzyme-protein, antigen-antibody, and hormone-receptor binding. A structural feature of an enzyme will attach to a specific structural feature of a protein.
1. Introduction. After Emil Fischer coined the lock-and-key picture for the reaction between enzymes and substrates [], it became a leading concept for the understanding of intermolecular interactions with proteins, and later for the rational design of drugs.With the advent of supramolecular chemistry the idea gained an enormous momentum, as chemists began to synthetize a large variety of host ...
The first requirement for any successful docking simulation is to define an active site or binding pocket as this is a critical step in structure-based drug design, ... Schematic of 'Combination Lock' hypothesis based on feature matching. Protein and Ligand's physicochemical properties are mapped and relevant binding features are extracted.
The lock and key model refers to an analogy used to describe the specific action of an enzyme with a substrate . During this process, the enzyme is acting as the lock and has a section called the active site which is where the reaction will take place, and the substrate, for instance a protein, is the key. In the same way that a key is paired ...
The induced fit model, in contrast to the lock-and-key paradigm, demonstrates that enzymes are very flexible structures. Emil Fischer proposed the lock and key model hypothesis in 1894, which demonstrates the great specificity of enzymes. It does not, however, explain the enzymes' ability to stabilise the transition state.
Lock and Key Model. The Lock and Key model is a theory of enzyme action hypothesized by Emil Fischer in 1899. According to Fischer, enzymes exhibit a high degree of specificity to the substances ...
The Lock-and-Key Hypothesis: Evolutionary and Biosystematic Interpretation of Insect Genitalia
A theory to explain the mechanism of enzymatic reactions, in which it is proposed that the enzyme and substrate (s) bind temporarily to form an enzyme-substrate complex. The binding site on the enzyme is known as the 'active site' and is structurally complementary to the substrate (s). Thus the enzyme and substrate (s) are said to fit ...
The rigorous definition of function given by Wright (80), "The function of X isZ means (a) X is there because it does Z and (b) Z is a ... The lock-and-key hypothesis purports to explain the ...
The lock & key model. The 'lock and key theory' is one simplified model that is used to explain enzyme action; The enzyme is like a lock, with the substrate(s) the keys that can fit into the active site of the enzyme with the two being a perfect fit; Diagram showing the lock and key model. Enzymes and substrates move about randomly in solution
1. Introduction. Two features of Locke's intellectual landscape are most salient for understanding his philosophy of science, one of which concerns the new science's methodology, and the other its content. First, then, is the new methodological approach to understanding the natural world. This approach is accompanied by profound shifts in ...
The lock and key hypothesis: The theory was postulated by Emil Fisher in 1898. According to the hypothesis, like a lock can be open by its key only, a substance possessing specific composition only can combine with the specific active site found in the specific enzyme's surface.
Partnerships with allies and across the services are key to maintaining the United States' edge amid an increasingly challenging national security backdrop, the military's top officers said.