Thank you for visiting nature.com. You are using a browser version with limited support for CSS. To obtain the best experience, we recommend you use a more up to date browser (or turn off compatibility mode in Internet Explorer). In the meantime, to ensure continued support, we are displaying the site without styles and JavaScript.
- View all journals
- Explore content
- About the journal
- Publish with us
- Sign up for alerts
- Review Article
- Published: 29 March 2022
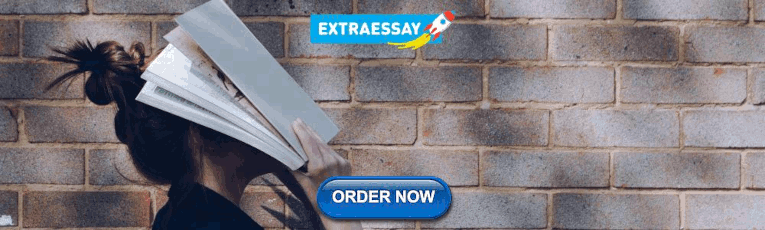
Music in the brain
- Peter Vuust ORCID: orcid.org/0000-0002-4908-735X 1 ,
- Ole A. Heggli ORCID: orcid.org/0000-0002-7461-0309 1 ,
- Karl J. Friston ORCID: orcid.org/0000-0001-7984-8909 2 &
- Morten L. Kringelbach ORCID: orcid.org/0000-0002-3908-6898 1 , 3 , 4
Nature Reviews Neuroscience volume 23 , pages 287–305 ( 2022 ) Cite this article
25k Accesses
93 Citations
278 Altmetric
Metrics details
- Neuroscience
Music is ubiquitous across human cultures — as a source of affective and pleasurable experience, moving us both physically and emotionally — and learning to play music shapes both brain structure and brain function. Music processing in the brain — namely, the perception of melody, harmony and rhythm — has traditionally been studied as an auditory phenomenon using passive listening paradigms. However, when listening to music, we actively generate predictions about what is likely to happen next. This enactive aspect has led to a more comprehensive understanding of music processing involving brain structures implicated in action, emotion and learning. Here we review the cognitive neuroscience literature of music perception. We show that music perception, action, emotion and learning all rest on the human brain’s fundamental capacity for prediction — as formulated by the predictive coding of music model. This Review elucidates how this formulation of music perception and expertise in individuals can be extended to account for the dynamics and underlying brain mechanisms of collective music making. This in turn has important implications for human creativity as evinced by music improvisation. These recent advances shed new light on what makes music meaningful from a neuroscientific perspective.
This is a preview of subscription content, access via your institution
Access options
Access Nature and 54 other Nature Portfolio journals
Get Nature+, our best-value online-access subscription
24,99 € / 30 days
cancel any time
Subscribe to this journal
Receive 12 print issues and online access
176,64 € per year
only 14,72 € per issue
Rent or buy this article
Prices vary by article type
Prices may be subject to local taxes which are calculated during checkout
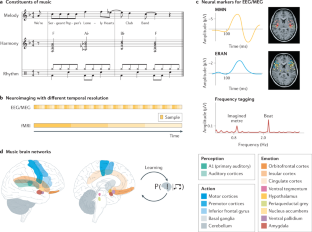
Similar content being viewed by others
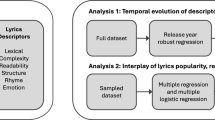
Song lyrics have become simpler and more repetitive over the last five decades
Emilia Parada-Cabaleiro, Maximilian Mayerl, … Eva Zangerle
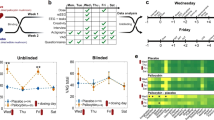
Microdosing with psilocybin mushrooms: a double-blind placebo-controlled study
Federico Cavanna, Stephanie Muller, … Enzo Tagliazucchi
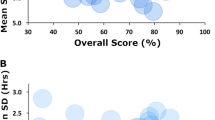
Sleep quality, duration, and consistency are associated with better academic performance in college students
Kana Okano, Jakub R. Kaczmarzyk, … Jeffrey C. Grossman
Zatorre, R. J., Chen, J. L. & Penhune, V. B. When the brain plays music: auditory–motor interactions in music perception and production. Nat. Rev. Neurosci. 8 , 547–558 (2007). A seminal review of auditory–motor coupling in music .
Article CAS PubMed Google Scholar
Koelsch, S. Toward a neural basis of music perception–a review and updated model. Front. Psychol. 2 , 110 (2011).
Article PubMed PubMed Central Google Scholar
Maes, P. J., Leman, M., Palmer, C. & Wanderley, M. M. Action-based effects on music perception. Front. Psychol. 4 , 1008 (2014).
Koelsch, S. Brain correlates of music-evoked emotions. Nat. Rev. Neurosci. 15 , 170–180 (2014). In this review, the author shows how music engages phylogenetically old reward networks in the brain to evoke emotions, and not merely subjective feelings .
Vuust, P. & Witek, M. A. Rhythmic complexity and predictive coding: a novel approach to modeling rhythm and meter perception in music. Front. Psychol. 5 , 1111 (2014).
Friston, K. The free-energy principle: a unified brain theory? Nat. Rev. Neurosci. 11 , 127–138 (2010). This review posits that several global brain theories may be unified by the free-energy principle .
Koelsch, S., Vuust, P. & Friston, K. Predictive processes and the peculiar case of music. Trends Cogn. Sci. 23 , 63–77 (2019). This review focuses specifically on predictive coding in music .
Article PubMed Google Scholar
Meyer, L. Emotion and Meaning in Music (Univ. of Chicago Press, 1956).
Lerdahl, F. & Jackendoff, R. A Generative Theory of Music (MIT Press, 1999).
Huron, D. Sweet Anticipation (MIT Press, 2006). In this book, Huron draws on evolutionary theory and statistical learning to propose a general theory of musical expectation .
Hansen, N. C. & Pearce, M. T. Predictive uncertainty in auditory sequence processing. Front. Psychol. https://doi.org/10.3389/fpsyg.2013.01008 (2014).
Vuust, P., Brattico, E., Seppanen, M., Naatanen, R. & Tervaniemi, M. The sound of music: differentiating musicians using a fast, musical multi-feature mismatch negativity paradigm. Neuropsychologia 50 , 1432–1443 (2012).
Altenmüller, E. O. How many music centers are in the brain? Ann. N. Y. Acad. Sci. 930 , 273–280 (2001).
Monelle, R. Linguistics and Semiotics in Music (Harwood Academic Publishers, 1992).
Rohrmeier, M. A. & Koelsch, S. Predictive information processing in music cognition. A critical review. Int. J. Psychophysiol. 83 , 164–175 (2012).
Vuust, P., Dietz, M. J., Witek, M. & Kringelbach, M. L. Now you hear it: a predictive coding model for understanding rhythmic incongruity. Ann. N. Y. Acad. Sci. https://doi.org/10.1111/nyas.13622 (2018).
Vuust, P., Ostergaard, L., Pallesen, K. J., Bailey, C. & Roepstorff, A. Predictive coding of music–brain responses to rhythmic incongruity. Cortex 45 , 80–92 (2009).
Vuust, P. & Frith, C. Anticipation is the key to understanding music and the effects of music on emotion. Behav. Brain Res. 31 , 599–600 (2008). This is the foundation for the PCM model used in this Review .
Google Scholar
Garrido, M. I., Sahani, M. & Dolan, R. J. Outlier responses reflect sensitivity to statistical structure in the human brain. PLoS Comput. Biol. 9 , e1002999 (2013).
Article CAS PubMed PubMed Central Google Scholar
Lumaca, M., Baggio, G., Brattico, E., Haumann, N. T. & Vuust, P. From random to regular: neural constraints on the emergence of isochronous rhythm during cultural transmission. Soc. Cogn. Affect. Neurosci. 13 , 877–888 (2018).
Quiroga-Martinez, D. R. et al. Musical prediction error responses similarly reduced by predictive uncertainty in musicians and non-musicians. Eur. J. Neurosci. https://doi.org/10.1111/ejn.14667 (2019).
Article Google Scholar
Koelsch, S., Schröger, E. & Gunter, T. C. Music matters: preattentive musicality of the human brain. Psychophysiology 39 , 38–48 (2002).
Koelsch, S., Schmidt, B.-h & Kansok, J. Effects of musical expertise on the early right anterior negativity: an event-related brain potential study. Psychophysiology 39 , 657–663 (2002).
Lumaca, M., Dietz, M. J., Hansen, N. C., Quiroga-Martinez, D. R. & Vuust, P. Perceptual learning of tone patterns changes the effective connectivity between Heschl’s gyrus and planum temporale. Hum. Brain Mapp. 42 , 941–952 (2020).
Lieder, F., Daunizeau, J., Garrido, M. I., Friston, K. J. & Stephan, K. E. Modelling trial-by-trial changes in the mismatch negativity. PLoS Comput. Biol. 9 , e1002911 (2013).
Wacongne, C., Changeux, J. P. & Dehaene, S. A neuronal model of predictive coding accounting for the mismatch negativity. J. Neurosci. 32 , 3665–3678 (2012).
Kiebel, S. J., Garrido, M. I. & Friston, K. J. Dynamic causal modelling of evoked responses: the role of intrinsic connections. Neuroimage 36 , 332–345 (2007).
Feldman, H. & Friston, K. J. Attention, uncertainty, and free-energy. Front. Hum. Neurosci. 4 , 215 (2010).
Cheung, V. K. M. et al. Uncertainty and surprise jointly predict musical pleasure and amygdala, hippocampus, and auditory cortex activity. Curr. Biol. 29 , 4084–4092 e4084 (2019). This fMRI study ties uncertainty and surprise to musical pleasure .
McDermott, J. H. & Oxenham, A. J. Music perception, pitch, and the auditory system. Curr. Opin. Neurobiol. 18 , 452–463 (2008).
Thoret, E., Caramiaux, B., Depalle, P. & McAdams, S. Learning metrics on spectrotemporal modulations reveals the perception of musical instrument timbre. Nat. Hum. Behav. 5 , 369–377 (2020).
Siedenburg, K. & McAdams, S. Four distinctions for the auditory “wastebasket” of timbre. Front. Psychol. 8 , 1747 (2017).
Bendor, D. & Wang, X. The neuronal representation of pitch in primate auditory cortex. Nature 436 , 1161–1165 (2005).
Zatorre, R. J. Pitch perception of complex tones and human temporal-lobe function. J. Acoustical Soc. Am. 84 , 566–572 (1988).
Article CAS Google Scholar
Warren, J. D., Uppenkamp, S., Patterson, R. D. & Griffiths, T. D. Separating pitch chroma and pitch height in the human brain. Proc. Natl Acad. Sci. USA 100 , 10038–10042 (2003). Using fMRI data, this study shows that pitch chroma is represented anterior to the primary auditory cortex, and pitch height is represented posterior to the primary auditory cortex .
Rauschecker, J. P. & Scott, S. K. Maps and streams in the auditory cortex: nonhuman primates illuminate human speech processing. Nat. Neurosci. 12 , 718–724 (2009).
Leaver, A. M., Van Lare, J., Zielinski, B., Halpern, A. R. & Rauschecker, J. P. Brain activation during anticipation of sound sequences. J. Neurosci. 29 , 2477–2485 (2009).
Houde, J. F. & Chang, E. F. The cortical computations underlying feedback control in vocal production. Curr. Opin. Neurobiol. 33 , 174–181 (2015).
Lee, Y. S., Janata, P., Frost, C., Hanke, M. & Granger, R. Investigation of melodic contour processing in the brain using multivariate pattern-based fMRI. Neuroimage 57 , 293–300 (2011).
Janata, P. et al. The cortical topography of tonal structures underlying Western music. Science 298 , 2167–2170 (2002).
Saffran, J. R., Aslin, R. N. & Newport, E. L. Statistical learning by 8-month-old infants. Science 274 , 1926–1928 (1996).
Saffran, J. R., Johnson, E. K., Aslin, R. N. & Newport, E. L. Statistical learning of tone sequences by human infants and adults. Cognition 70 , 27–52 (1999).
Krumhansl, C. L. Perceptual structures for tonal music. Music. Percept. 1 , 28–62 (1983).
Margulis, E. H. A model of melodic expectation. Music. Percept. 22 , 663–714 (2005).
Temperley, D. A probabilistic model of melody perception. Cogn. Sci. 32 , 418–444 (2008).
Pearce, M. T. & Wiggins, G. A. Auditory expectation: the information dynamics of music perception and cognition. Top. Cogn. Sci. 4 , 625–652 (2012).
Sears, D. R. W., Pearce, M. T., Caplin, W. E. & McAdams, S. Simulating melodic and harmonic expectations for tonal cadences using probabilistic models. J. N. Music. Res. 47 , 29–52 (2018).
Näätänen, R., Gaillard, A. W. & Mäntysalo, S. Early selective-attention effect on evoked potential reinterpreted. Acta Psychol. 42 , 313–329 (1978).
Näätänen, R., Paavilainen, P., Rinne, T. & Alho, K. The mismatch negativity (MMN) in basic research of central auditory processing: a review. Clin. Neurophysiol. 118 , 2544–2590 (2007). This classic review covers three decades of MMN research to understand auditory perception .
Wallentin, M., Nielsen, A. H., Friis-Olivarius, M., Vuust, C. & Vuust, P. The Musical Ear Test, a new reliable test for measuring musical competence. Learn. Individ. Differ. 20 , 188–196 (2010).
Tervaniemi, M. et al. Top-down modulation of auditory processing: effects of sound context, musical expertise and attentional focus. Eur. J. Neurosci. 30 , 1636–1642 (2009).
Burunat, I. et al. The reliability of continuous brain responses during naturalistic listening to music. Neuroimage 124 , 224–231 (2016).
Burunat, I. et al. Action in perception: prominent visuo-motor functional symmetry in musicians during music listening. PLoS ONE 10 , e0138238 (2015).
Article PubMed PubMed Central CAS Google Scholar
Alluri, V. et al. Large-scale brain networks emerge from dynamic processing of musical timbre, key and rhythm. Neuroimage 59 , 3677–3689 (2012). A free-listening fMRI study showing brain networks involved in perception of distinct acoustical features of music .
Halpern, A. R. & Zatorre, R. J. When that tune runs through your head: a PET investigation of auditory imagery for familiar melodies. Cereb. Cortex 9 , 697–704 (1999).
Herholz, S. C., Halpern, A. R. & Zatorre, R. J. Neuronal correlates of perception, imagery, and memory for familiar tunes. J. Cogn. Neurosci. 24 , 1382–1397 (2012).
Pallesen, K. J. et al. Emotion processing of major, minor, and dissonant chords: a functional magnetic resonance imaging study. Ann. N. Y. Acad. Sci. 1060 , 450–453 (2005).
McPherson, M. J. et al. Perceptual fusion of musical notes by native Amazonians suggests universal representations of musical intervals. Nat. Commun. 11 , 2786 (2020).
Helmholtz H. L. F. On the Sensations of Tone as a Physiological Basis for the Theory of Music (Cambridge Univ. Press, 1954).
Vassilakis, P. N. & Kendall, R. A. in Human Vision and Electronic Imaging XV . 75270O (International Society for Optics and Photonics, 2010).
Plomp, R. & Levelt, W. J. M. Tonal consonance and critical bandwidth. J. Acoustical Soc. Am. 38 , 548–560 (1965).
McDermott, J. H., Schultz, A. F., Undurraga, E. A. & Godoy, R. A. Indifference to dissonance in native Amazonians reveals cultural variation in music perception. Nature 535 , 547–550 (2016). An ethnomusicology study showing that consonance preference may be absent in people with minimal exposure to Western music .
Mehr, S. A. et al. Universality and diversity in human song. Science https://doi.org/10.1126/science.aax0868 (2019).
Patel, A. D., Gibson, E., Ratner, J., Besson, M. & Holcomb, P. J. Processing syntactic relations in language and music: an event-related potential study. J. Cogn. Neurosci. 10 , 717–733 (1998). This classic study compares responses to syntactic incongruities in both language and Western tonal music .
Janata, P. The neural architecture of music-evoked autobiographical memories. Cereb. Cortex 19 , 2579–2594 (2009).
Maess, B., Koelsch, S., Gunter, T. C. & Friederici, A. D. Musical syntax is processed in Broca’s area: an MEG study. Nat. Neurosci. 4 , 540–545 (2001).
Koelsch, S. et al. Differentiating ERAN and MMN: an ERP study. Neuroreport 12 , 1385–1389 (2001). Using EEG, the authors show that ERAN and MMN reflect different cognitive mechanisms .
Loui, P., Grent-‘t-Jong, T., Torpey, D. & Woldorff, M. Effects of attention on the neural processing of harmonic syntax in Western music. Cogn. Brain Res. 25 , 678–687 (2005).
Koelsch, S., Fritz, T., Schulze, K., Alsop, D. & Schlaug, G. Adults and children processing music: an fMRI study. Neuroimage 25 , 1068–1076 (2005).
Tillmann, B., Janata, P. & Bharucha, J. J. Activation of the inferior frontal cortex in musical priming. Ann. N. Y. Acad. Sci. 999 , 209–211 (2003).
Garza-Villarreal, E. A., Brattico, E., Leino, S., Ostergaard, L. & Vuust, P. Distinct neural responses to chord violations: a multiple source analysis study. Brain Res. 1389 , 103–114 (2011).
Leino, S., Brattico, E., Tervaniemi, M. & Vuust, P. Representation of harmony rules in the human brain: further evidence from event-related potentials. Brain Res. 1142 , 169–177 (2007).
Sammler, D. et al. Co-localizing linguistic and musical syntax with intracranial EEG. Neuroimage 64 , 134–146 (2013).
Loui, P., Wessel, D. L. & Hudson Kam, C. L. Humans rapidly learn grammatical structure in a new musical scale. Music. Percept. 27 , 377–388 (2010).
Loui, P., Wu, E. H., Wessel, D. L. & Knight, R. T. A generalized mechanism for perception of pitch patterns. J. Neurosci. 29 , 454–459 (2009).
Cheung, V. K. M., Meyer, L., Friederici, A. D. & Koelsch, S. The right inferior frontal gyrus processes nested non-local dependencies in music. Sci. Rep. 8 , 3822 (2018).
Haueisen, J. & Knosche, T. R. Involuntary motor activity in pianists evoked by music perception. J. Cogn. Neurosci. 13 , 786–792 (2001).
Bangert, M. et al. Shared networks for auditory and motor processing in professional pianists: evidence from fMRI conjunction. Neuroimage 30 , 917–926 (2006).
Baumann, S. et al. A network for audio-motor coordination in skilled pianists and non-musicians. Brain Res. 1161 , 65–78 (2007).
Lahav, A., Saltzman, E. & Schlaug, G. Action representation of sound: audiomotor recognition network while listening to newly acquired actions. J. Neurosci. 27 , 308–314 (2007).
Bianco, R. et al. Neural networks for harmonic structure in music perception and action. Neuroimage 142 , 454–464 (2016).
Eerola, T., Vuoskoski, J. K., Peltola, H.-R., Putkinen, V. & Schäfer, K. An integrative review of the enjoyment of sadness associated with music. Phys. Life Rev. 25 , 100–121 (2018).
Huron, D. M. D. The harmonic minor scale provides an optimum way of reducing average melodic interval size, consistent with sad affect cues. Empir. Musicol. Rev. 7 , 15 (2012).
Huron, D. A comparison of average pitch height and interval size in major-and minor-key themes: evidence consistent with affect-related pitch prosody. 3 , 59-63 (2008).
Juslin, P. N. & Laukka, P. Communication of emotions in vocal expression and music performance: different channels, same code? Psychol. Bull. 129 , 770 (2003).
Fritz, T. et al. Universal recognition of three basic emotions in music. Curr. Biol. 19 , 573–576 (2009).
London, J. Hearing in Time: Psychological Aspects of Musical Meter (Oxford Univ. Press, 2012).
Honing, H. Without it no music: beat induction as a fundamental musical trait. Ann. N. Y. Acad. Sci. 1252 , 85–91 (2012).
Hickok, G., Farahbod, H. & Saberi, K. The rhythm of perception: entrainment to acoustic rhythms induces subsequent perceptual oscillation. Psychol. Sci. 26 , 1006–1013 (2015).
Yabe, H., Tervaniemi, M., Reinikainen, K. & Näätänen, R. Temporal window of integration revealed by MMN to sound omission. Neuroreport 8 , 1971–1974 (1997).
Andreou, L.-V., Griffiths, T. D. & Chait, M. Sensitivity to the temporal structure of rapid sound sequences — an MEG study. Neuroimage 110 , 194–204 (2015).
Jongsma, M. L., Meeuwissen, E., Vos, P. G. & Maes, R. Rhythm perception: speeding up or slowing down affects different subcomponents of the ERP P3 complex. Biol. Psychol. 75 , 219–228 (2007).
Graber, E. & Fujioka, T. Endogenous expectations for sequence continuation after auditory beat accelerations and decelerations revealed by P3a and induced beta-band responses. Neuroscience 413 , 11–21 (2019).
Brochard, R., Abecasis, D., Potter, D., Ragot, R. & Drake, C. The “ticktock” of our internal clock: direct brain evidence of subjective accents in isochronous sequences. Psychol. Sci. 14 , 362–366 (2003).
Lerdahl, F. & Jackendoff, R. An overview of hierarchical structure in music. Music. Percept. 1 , 229–252 (1983).
Large, E. W. & Kolen, J. F. Resonance and the perception of musical meter. Connect. Sci. 6 , 177–208 (1994).
Large, E. W. & Jones, M. R. The dynamics of attending: how people track time-varying events. Psychol. Rev. 106 , 119–159 (1999).
Cutietta, R. A. & Booth, G. D. The influence of metre, mode, interval type and contour in repeated melodic free-recall. Psychol. Music 24 , 222–236 (1996).
Smith, K. C. & Cuddy, L. L. Effects of metric and harmonic rhythm on the detection of pitch alterations in melodic sequences. J. Exp. Psychol. 15 , 457–471 (1989).
CAS Google Scholar
Palmer, C. & Krumhansl, C. L. Mental representations for musical meter. J. Exp. Psychol. 16 , 728–741 (1990).
Einarson, K. M. & Trainor, L. J. Hearing the beat: young children’s perceptual sensitivity to beat alignment varies according to metric structure. Music. Percept. 34 , 56–70 (2016).
Large, E. W., Herrera, J. A. & Velasco, M. J. Neural networks for beat perception in musical rhythm. Front. Syst. Neurosci. 9 , 159 (2015).
Nozaradan, S., Peretz, I., Missal, M. & Mouraux, A. Tagging the neuronal entrainment to beat and meter. J. Neurosci. 31 , 10234–10240 (2011).
Nozaradan, S., Peretz, I. & Mouraux, A. Selective neuronal entrainment to the beat and meter embedded in a musical rhythm. J. Neurosci. 32 , 17572–17581 (2012).
Nozaradan, S., Schonwiesner, M., Keller, P. E., Lenc, T. & Lehmann, A. Neural bases of rhythmic entrainment in humans: critical transformation between cortical and lower-level representations of auditory rhythm. Eur. J. Neurosci. 47 , 321–332 (2018).
Lenc, T., Keller, P. E., Varlet, M. & Nozaradan, S. Neural and behavioral evidence for frequency-selective context effects in rhythm processing in humans. Cereb. Cortex Commun. https://doi.org/10.1093/texcom/tgaa037 (2020).
Jacoby, N. & McDermott, J. H. Integer ratio priors on musical rhythm revealed cross-culturally by iterated reproduction. Curr. Biol. 27 , 359–370 (2017).
Hannon, E. E. & Trehub, S. E. Metrical categories in infancy and adulthood. Psychol. Sci. 16 , 48–55 (2005).
Hannon, E. E. & Trehub, S. E. Tuning in to musical rhythms: infants learn more readily than adults. Proc. Natl Acad. Sci. USA 102 , 12639–12643 (2005).
Vuust, P. et al. To musicians, the message is in the meter pre-attentive neuronal responses to incongruent rhythm are left-lateralized in musicians. Neuroimage 24 , 560–564 (2005).
Grahn, J. A. & Brett, M. Rhythm and beat perception in motor areas of the brain. J. Cogn. Neurosci. 19 , 893–906 (2007). This fMRI study investigates participants listening to rhythms of varied complexity .
Toiviainen, P., Burunat, I., Brattico, E., Vuust, P. & Alluri, V. The chronnectome of musical beat. Neuroimage 216 , 116191 (2019).
Chen, J. L., Penhune, V. B. & Zatorre, R. J. Moving on time: brain network for auditory-motor synchronization is modulated by rhythm complexity and musical training. J. Cogn. Neurosci. 20 , 226–239 (2008).
Levitin, D. J., Grahn, J. A. & London, J. The psychology of music: rhythm and movement. Annu. Rev. Psychol. 69 , 51–75 (2018).
Winkler, I., Haden, G. P., Ladinig, O., Sziller, I. & Honing, H. Newborn infants detect the beat in music. Proc. Natl Acad. Sci. USA 106 , 2468–2471 (2009).
Phillips-Silver, J. & Trainor, L. J. Feeling the beat: movement influences infant rhythm perception. Science 308 , 1430–1430 (2005).
Cirelli, L. K., Trehub, S. E. & Trainor, L. J. Rhythm and melody as social signals for infants. Ann. N. Y. Acad. Sci. https://doi.org/10.1111/nyas.13580 (2018).
Cirelli, L. K., Einarson, K. M. & Trainor, L. J. Interpersonal synchrony increases prosocial behavior in infants. Dev. Sci. 17 , 1003–1011 (2014).
Repp, B. H. Sensorimotor synchronization: a review of the tapping literature. Psychon. Bull. Rev. 12 , 969–992 (2005).
Repp, B. H. & Su, Y. H. Sensorimotor synchronization: a review of recent research (2006-2012). Psychon. Bull. Rev. 20 , 403–452 (2013). This review, and Repp (2005), succinctly covers the field of sensorimotor synchronization .
Zarco, W., Merchant, H., Prado, L. & Mendez, J. C. Subsecond timing in primates: comparison of interval production between human subjects and rhesus monkeys. J. Neurophysiol. 102 , 3191–3202 (2009).
Honing, H., Bouwer, F. L., Prado, L. & Merchant, H. Rhesus monkeys ( Macaca mulatta ) sense isochrony in rhythm, but not the beat: additional support for the gradual audiomotor evolution hypothesis. Front. Neurosci. 12 , 475 (2018).
Hattori, Y. & Tomonaga, M. Rhythmic swaying induced by sound in chimpanzees (Pan troglodytes). Proc. Natl Acad. Sci. USA 117 , 936–942 (2020).
Danielsen, A. Presence and Pleasure. The Funk Grooves of James Brown and Parliament (Wesleyan Univ. Press, 2006).
Madison, G., Gouyon, F., Ullen, F. & Hornstrom, K. Modeling the tendency for music to induce movement in humans: first correlations with low-level audio descriptors across music genres. J. Exp. Psychol. Hum. Percept. Perform. 37 , 1578–1594 (2011).
Stupacher, J., Hove, M. J., Novembre, G., Schutz-Bosbach, S. & Keller, P. E. Musical groove modulates motor cortex excitability: a TMS investigation. Brain Cogn. 82 , 127–136 (2013).
Janata, P., Tomic, S. T. & Haberman, J. M. Sensorimotor coupling in music and the psychology of the groove. J. Exp. Psychol. 141 , 54 (2012). Using a systematic approach, this multiple-studies article shows that the concept of groove can be widely understood as a pleasurable drive towards action .
Witek, M. A. et al. A critical cross-cultural study of sensorimotor and groove responses to syncopation among Ghanaian and American university students and staff. Music. Percept. 37 , 278–297 (2020).
Friston, K., Mattout, J. & Kilner, J. Action understanding and active inference. Biol. Cybern. 104 , 137–160 (2011).
Longuet-Higgins, H. C. & Lee, C. S. The rhythmic interpretation of monophonic music. Music. Percept. 1 , 18 (1984).
Sioros, G., Miron, M., Davies, M., Gouyon, F. & Madison, G. Syncopation creates the sensation of groove in synthesized music examples. Front. Psychol. 5 , 1036 (2014).
Witek, M. A., Clarke, E. F., Wallentin, M., Kringelbach, M. L. & Vuust, P. Syncopation, body-movement and pleasure in groove music. PLoS ONE 9 , e94446 (2014).
Kowalewski, D. A., Kratzer, T. M. & Friedman, R. S. Social music: investigating the link between personal liking and perceived groove. Music. Percept. 37 , 339–346 (2020).
Bowling, D. L., Ancochea, P. G., Hove, M. J. & Tecumseh Fitch, W. Pupillometry of groove: evidence for noradrenergic arousal in the link between music and movement. Front. Neurosci. 13 , 1039 (2019).
Matthews, T. E., Witek, M. A. G., Heggli, O. A., Penhune, V. B. & Vuust, P. The sensation of groove is affected by the interaction of rhythmic and harmonic complexity. PLoS ONE 14 , e0204539 (2019).
Matthews, T. E., Witek, M. A., Lund, T., Vuust, P. & Penhune, V. B. The sensation of groove engages motor and reward networks. Neuroimage 214 , 116768 (2020). This fMRI study shows that the sensation of groove engages both motor and reward networks in the brain .
Vaquero, L., Ramos-Escobar, N., François, C., Penhune, V. & Rodríguez-Fornells, A. White-matter structural connectivity predicts short-term melody and rhythm learning in non-musicians. Neuroimage 181 , 252–262 (2018).
Zatorre, R. J., Halpern, A. R., Perry, D. W., Meyer, E. & Evans, A. C. Hearing in the mind’s ear: a PET investigation of musical imagery and perception. J. Cogn. Neurosci. 8 , 29–46 (1996).
Benadon, F. Meter isn’t everything: the case of a timeline-oriented Cuban polyrhythm. N. Ideas Psychol. 56 , 100735 (2020).
London, J., Polak, R. & Jacoby, N. Rhythm histograms and musical meter: a corpus study of Malian percussion music. Psychon. Bull. Rev. 24 , 474–480 (2017).
Huron, D. Is music an evolutionary adaptation? Ann. N. Y. Acad. Sci. 930 , 43–61 (2001).
Koelsch, S. Towards a neural basis of music-evoked emotions. Trends Cogn. Sci. 14 , 131–137 (2010).
Eerola, T. & Vuoskoski, J. K. A comparison of the discrete and dimensional models of emotion in music. Psychol. Music. 39 , 18–49 (2010).
Lonsdale, A. J. & North, A. C. Why do we listen to music? A uses and gratifications analysis. Br. J. Psychol. 102 , 108–134 (2011).
Juslin, P. N. & Laukka, P. Expression, perception, and induction of musical emotions: a review and a questionnaire study of everyday listening. J. N. Music. Res. 33 , 217–238 (2004).
Huron, D. Why is sad music pleasurable? A possible role for prolactin. Music. Sci. 15 , 146–158 (2011).
Brattico, E. et al. It’s sad but I like it: the neural dissociation between musical emotions and liking in experts and laypersons. Front. Hum. Neurosci. 9 , 676 (2015).
PubMed Google Scholar
Sachs, M. E., Damasio, A. & Habibi, A. Unique personality profiles predict when and why sad music is enjoyed. Psychol. Music https://doi.org/10.1177/0305735620932660 (2020).
Sachs, M. E., Habibi, A., Damasio, A. & Kaplan, J. T. Dynamic intersubject neural synchronization reflects affective responses to sad music. Neuroimage 218 , 116512 (2020).
Juslin, P. N. & Vastfjall, D. Emotional responses to music: the need to consider underlying mechanisms. Behav. Brain Sci. 31 , 559–575 (2008). Using a novel theoretical framework, the authors propose that the mechanisms that evoke emotions from music are not unique to music .
Rickard, N. S. Intense emotional responses to music: a test of the physiological arousal hypothesis. Psychol. Music. 32 , 371–388 (2004).
Cowen, A. S., Fang, X., Sauter, D. & Keltner, D. What music makes us feel: at least 13 dimensions organize subjective experiences associated with music across different cultures. Proc. Natl Acad. Sci. USA 117 , 1924–1934 (2020).
Argstatter, H. Perception of basic emotions in music: culture-specific or multicultural? Psychol. Music. 44 , 674–690 (2016).
Stevens, C. J. Music perception and cognition: a review of recent cross-cultural research. Top. Cogn. Sci. 4 , 653–667 (2012).
Pearce, M. Cultural distance: a computational approach to exploring cultural influences on music cognition. in Oxford Handbook of Music and the Brain Vol. 31 (Oxford Univ. Press, 2018).
van der Weij, B., Pearce, M. T. & Honing, H. A probabilistic model of meter perception: simulating enculturation. Front. Psychol. 8 , 824 (2017).
Kringelbach, M. L. & Berridge, K. C. Towards a functional neuroanatomy of pleasure and happiness. Trends Cogn. Sci. 13 , 479–487 (2009).
Blood, A. J. & Zatorre, R. J. Intensely pleasurable responses to music correlate with activity in brain regions implicated in reward and emotion. Proc. Natl Acad. Sci. USA 98 , 11818–11823 (2001). This seminal positron emission tomography study shows that the experience of musical chills correlates with activity in the reward system .
Salimpoor, V. N. & Zatorre, R. J. Complex cognitive functions underlie aesthetic emotions: comment on “From everyday emotions to aesthetic emotions: towards a unified theory of musical emotions” by Patrik N. Juslin. Phys. Life Rev. 10 , 279–280 (2013).
Salimpoor, V. N. et al. Interactions between the nucleus accumbens and auditory cortices predict music reward value. Science 340 , 216–219 (2013).
Salimpoor, V. N., Benovoy, M., Larcher, K., Dagher, A. & Zatorre, R. J. Anatomically distinct dopamine release during anticipation and experience of peak emotion to music. Nat. Neurosci. 14 , 257–262 (2011).
Salimpoor, V. N., Benovoy, M., Longo, G., Cooperstock, J. R. & Zatorre, R. J. The rewarding aspects of music listening are related to degree of emotional arousal. PLoS ONE 4 , e7487 (2009).
Mas-Herrero, E., Zatorre, R. J., Rodriguez-Fornells, A. & Marco-Pallares, J. Dissociation between musical and monetary reward responses in specific musical anhedonia. Curr. Biol. 24 , 699–704 (2014).
Martinez-Molina, N., Mas-Herrero, E., Rodriguez-Fornells, A., Zatorre, R. J. & Marco-Pallares, J. Neural correlates of specific musical anhedonia. Proc. Natl Acad. Sci. USA 113 , E7337–E7345 (2016).
Gebauer, L. K., M., L. & Vuust, P. Musical pleasure cycles: the role of anticipation and dopamine. Psychomusicology 22 , 16 (2012).
Shany, O. et al. Surprise-related activation in the nucleus accumbens interacts with music-induced pleasantness. Soc. Cogn. Affect. Neurosci. 14 , 459–470 (2019).
Gold, B. P., Pearce, M. T., Mas-Herrero, E., Dagher, A. & Zatorre, R. J. Predictability and uncertainty in the pleasure of music: a reward for learning? J. Neurosci. 39 , 9397–9409 (2019).
Swaminathan, S. & Schellenberg, E. G. Current emotion research in music psychology. Emot. Rev. 7 , 189–197 (2015).
Madison, G. & Schiölde, G. Repeated listening increases the liking for music regardless of its complexity: implications for the appreciation and aesthetics of music. Front. Neurosci. 11 , 147 (2017).
Corrigall, K. A. & Schellenberg, E. G. Liking music: genres, contextual factors, and individual differences. in Art, Aesthetics, and the Brain (Oxford Univ. Press, 2015).
Zentner, A. Measuring the effect of file sharing on music purchases. J. Law Econ. 49 , 63–90 (2006).
Rentfrow, P. J. & Gosling, S. D. The do re mi’s of everyday life: the structure and personality correlates of music preferences. J. Pers. Soc. Psychol. 84 , 1236–1256 (2003).
Vuust, P. et al. Personality influences career choice: sensation seeking in professional musicians. Music. Educ. Res. 12 , 219–230 (2010).
Rohrmeier, M. & Rebuschat, P. Implicit learning and acquisition of music. Top. Cogn. Sci. 4 , 525–553 (2012).
Münthe, T. F., Altenmüller, E. & Jäncke, L. The musician’s brain as a model of neuroplasticity. Nat. Rev. Neurosci. 3 , 1–6 (2002). This review highlights how professional musicians represent an ideal model for investigating neuroplasticity .
Habibi, A. et al. Childhood music training induces change in micro and macroscopic brain structure: results from a longitudinal study. Cereb. Cortex 28 , 4336–4347 (2018).
Schlaug, G., Jancke, L., Huang, Y., Staiger, J. F. & Steinmetz, H. Increased corpus callosum size in musicians. Neuropsychologia 33 , 1047–1055 (1995).
Baer, L. H. et al. Regional cerebellar volumes are related to early musical training and finger tapping performance. Neuroimage 109 , 130–139 (2015).
Kleber, B. et al. Voxel-based morphometry in opera singers: increased gray-matter volume in right somatosensory and auditory cortices. Neuroimage 133 , 477–483 (2016).
Gaser, C. & Schlaug, G. Brain structures differ between musicians and non-musicians. J. Neurosci. 23 , 9240–9245 (2003). Using a morphometric technique, this study shows a grey matter volume difference in multiple brain regions between professional musicians and a matched control group of amateur musicians and non-musicians .
Sluming, V. et al. Voxel-based morphometry reveals increased gray matter density in Broca’s area in male symphony orchestra musicians. Neuroimage 17 , 1613–1622 (2002).
Palomar-García, M.-Á., Zatorre, R. J., Ventura-Campos, N., Bueichekú, E. & Ávila, C. Modulation of functional connectivity in auditory–motor networks in musicians compared with nonmusicians. Cereb. Cortex 27 , 2768–2778 (2017).
Schneider, P. et al. Morphology of Heschl’s gyrus reflects enhanced activation in the auditory cortex of musicians. Nat. Neurosci. 5 , 688–694 (2002).
Bengtsson, S. L. et al. Extensive piano practicing has regionally specific effects on white matter development. Nat. Neurosci. 8 , 1148–1150 (2005).
Zamorano, A. M., Cifre, I., Montoya, P., Riquelme, I. & Kleber, B. Insula-based networks in professional musicians: evidence for increased functional connectivity during resting state fMRI. Hum. Brain Mapp. 38 , 4834–4849 (2017).
Kraus, N. & Chandrasekaran, B. Music training for the development of auditory skills. Nat. Rev. Neurosci. 11 , 599–605 (2010).
Koelsch, S., Schröger, E. & Tervaniemi, M. Superior pre-attentive auditory processing in musicians. Neuroreport 10 , 1309–1313 (1999).
Münte, T. F., Kohlmetz, C., Nager, W. & Altenmüller, E. Superior auditory spatial tuning in conductors. Nature 409 , 580 (2001).
Seppänen, M., Brattico, E. & Tervaniemi, M. Practice strategies of musicians modulate neural processing and the learning of sound-patterns. Neurobiol. Learn. Mem. 87 , 236–247 (2007).
Guillot, A. et al. Functional neuroanatomical networks associated with expertise in motor imagery. Neuroimage 41 , 1471–1483 (2008).
Bianco, R., Novembre, G., Keller, P. E., Villringer, A. & Sammler, D. Musical genre-dependent behavioural and EEG signatures of action planning. a comparison between classical and jazz pianists. Neuroimage 169 , 383–394 (2018).
Vuust, P., Brattico, E., Seppänen, M., Näätänen, R. & Tervaniemi, M. Practiced musical style shapes auditory skills. Ann. N. Y. Acad. Sci. 1252 , 139–146 (2012).
Bangert, M. & Altenmüller, E. O. Mapping perception to action in piano practice: a longitudinal DC-EEG study. BMC Neurosci. 4 , 26 (2003).
Li, Q. et al. Musical training induces functional and structural auditory-motor network plasticity in young adults. Hum. Brain Mapp. 39 , 2098–2110 (2018).
Herholz, S. C., Coffey, E. B. J., Pantev, C. & Zatorre, R. J. Dissociation of neural networks for predisposition and for training-related plasticity in auditory-motor learning. Cereb. Cortex 26 , 3125–3134 (2016).
Putkinen, V., Tervaniemi, M. & Huotilainen, M. Musical playschool activities are linked to faster auditory development during preschool-age: a longitudinal ERP study. Sci. Rep. 9 , 11310–11310 (2019).
Putkinen, V., Tervaniemi, M., Saarikivi, K., Ojala, P. & Huotilainen, M. Enhanced development of auditory change detection in musically trained school-aged children: a longitudinal event-related potential study. Dev. Sci. 17 , 282–297 (2014).
Jentschke, S. & Koelsch, S. Musical training modulates the development of syntax processing in children. Neuroimage 47 , 735–744 (2009).
Chobert, J., François, C., Velay, J. L. & Besson, M. Twelve months of active musical training in 8-to 10-year-old children enhances the preattentive processing of syllabic duration and voice onset time. Cereb. Cortex 24 , 956–967 (2014).
Moreno, S. et al. Musical training influences linguistic abilities in 8-year-old children: more evidence for brain plasticity. Cereb. Cortex 19 , 712–723 (2009).
Putkinen, V., Huotilainen, M. & Tervaniemi, M. Neural encoding of pitch direction is enhanced in musically trained children and is related to reading skills. Front. Psychol. 10 , 1475 (2019).
Wong, P. C., Skoe, E., Russo, N. M., Dees, T. & Kraus, N. Musical experience shapes human brainstem encoding of linguistic pitch patterns. Nat. Neurosci. 10 , 420–422 (2007).
Virtala, P. & Partanen, E. Can very early music interventions promote at-risk infants’ development? Ann. N. Y. Acad. Sci. 1423 , 92–101 (2018).
Flaugnacco, E. et al. Music training increases phonological awareness and reading skills in developmental dyslexia: a randomized control trial. PLoS ONE 10 , e0138715 (2015).
Fiveash, A. et al. A stimulus-brain coupling analysis of regular and irregular rhythms in adults with dyslexia and controls. Brain Cogn. 140 , 105531 (2020).
Schellenberg, E. G. Correlation = causation? music training, psychology, and neuroscience. Psychol. Aesthet. Creat. Arts 14 , 475–480 (2019).
Sala, G. & Gobet, F. Cognitive and academic benefits of music training with children: a multilevel meta-analysis. Mem. Cogn. 48 , 1429–1441 (2020).
Saffran, J. R. Musical learning and language development. Ann. N. Y. Acad. Sci. 999 , 397–401 (2003).
Friston, K. The free-energy principle: a rough guide to the brain? Trends Cogn. Sci. 13 , 293–301 (2009).
Pearce, M. T. Statistical learning and probabilistic prediction in music cognition: mechanisms of stylistic enculturation. Ann. N. Y. Acad. Sci. 1423 , 378–395 (2018).
Article PubMed Central Google Scholar
Novembre, G., Knoblich, G., Dunne, L. & Keller, P. E. Interpersonal synchrony enhanced through 20 Hz phase-coupled dual brain stimulation. Soc. Cogn. Affect. Neurosci. 12 , 662–670 (2017).
Konvalinka, I. et al. Frontal alpha oscillations distinguish leaders from followers: multivariate decoding of mutually interacting brains. Neuroimage 94C , 79–88 (2014).
Novembre, G., Mitsopoulos, Z. & Keller, P. E. Empathic perspective taking promotes interpersonal coordination through music. Sci. Rep. 9 , 12255 (2019).
Wolpert, D. M., Ghahramani, Z. & Jordan, M. I. An internal model for sensorimotor integration. Science 269 , 1880–1882 (1995).
Patel, A. D. & Iversen, J. R. The evolutionary neuroscience of musical beat perception: the action simulation for auditory prediction (ASAP) hypothesis. Front. Syst. Neurosci. 8 , 57 (2014).
Sebanz, N. & Knoblich, G. Prediction in joint action: what, when, and where. Top. Cogn. Sci. 1 , 353–367 (2009).
Friston, K. J. & Frith, C. D. Active inference, communication and hermeneutics. Cortex 68 , 129–143 (2015). This article proposes a link between active inference, communication and hermeneutics .
Konvalinka, I., Vuust, P., Roepstorff, A. & Frith, C. D. Follow you, follow me: continuous mutual prediction and adaptation in joint tapping. Q. J. Exp. Psychol. 63 , 2220–2230 (2010).
Wing, A. M. & Kristofferson, A. B. Response delays and the timing of discrete motor responses. Percept. Psychophys. 14 , 5–12 (1973).
Repp, B. H. & Keller, P. E. Sensorimotor synchronization with adaptively timed sequences. Hum. Mov. Sci. 27 , 423–456 (2008).
Vorberg, D. & Schulze, H.-H. Linear phase-correction in synchronization: predictions, parameter estimation, and simulations. J. Math. Psychol. 46 , 56–87 (2002).
Novembre, G., Sammler, D. & Keller, P. E. Neural alpha oscillations index the balance between self-other integration and segregation in real-time joint action. Neuropsychologia 89 , 414–425 (2016). Using dual-EEG, the authors propose alpha oscillations as a candidate for regulating the balance between internal and external information in joint action .
Keller, P. E., Knoblich, G. & Repp, B. H. Pianists duet better when they play with themselves: on the possible role of action simulation in synchronization. Conscious. Cogn. 16 , 102–111 (2007).
Fairhurst, M. T., Janata, P. & Keller, P. E. Leading the follower: an fMRI investigation of dynamic cooperativity and leader-follower strategies in synchronization with an adaptive virtual partner. Neuroimage 84 , 688–697 (2014).
Heggli, O. A., Konvalinka, I., Kringelbach, M. L. & Vuust, P. Musical interaction is influenced by underlying predictive models and musical expertise. Sci. Rep. 9 , 1–13 (2019).
Heggli, O. A., Cabral, J., Konvalinka, I., Vuust, P. & Kringelbach, M. L. A Kuramoto model of self-other integration across interpersonal synchronization strategies. PLoS Comput. Biol. 15 , e1007422 (2019).
Heggli, O. A. et al. Transient brain networks underlying interpersonal strategies during synchronized action. Soc. Cogn. Affect. Neurosci. 16 , 19–30 (2020). This EEG study shows that differences in interpersonal synchronization are reflected by activity in a temporoparietal network .
Patel, A. D. Music, Language, and the Brain (Oxford Univ. Press, 2006).
Molnar-Szakacs, I. & Overy, K. Music and mirror neurons: from motion to ‘e’motion. Soc. Cogn. Affect. Neurosci. 1 , 235–241 (2006).
Beaty, R. E., Benedek, M., Silvia, P. J. & Schacter, D. L. Creative cognition and brain network dynamics. Trends Cogn. Sci. 20 , 87–95 (2016).
Limb, C. J. & Braun, A. R. Neural substrates of spontaneous musical performance: an FMRI study of jazz improvisation. PLoS ONE 3 , e1679 (2008).
Liu, S. et al. Neural correlates of lyrical improvisation: an FMRI study of freestyle rap. Sci. Rep. 2 , 834 (2012).
Rosen, D. S. et al. Dual-process contributions to creativity in jazz improvisations: an SPM-EEG study. Neuroimage 213 , 116632 (2020).
Boasen, J., Takeshita, Y., Kuriki, S. & Yokosawa, K. Spectral-spatial differentiation of brain activity during mental imagery of improvisational music performance using MEG. Front. Hum. Neurosci. 12 , 156 (2018).
Berkowitz, A. L. & Ansari, D. Generation of novel motor sequences: the neural correlates of musical improvisation. Neuroimage 41 , 535–543 (2008).
Loui, P. Rapid and flexible creativity in musical improvisation: review and a model. Ann. N. Y. Acad. Sci. 1423 , 138–145 (2018).
Beaty, R. E. The neuroscience of musical improvisation. Neurosci. Biobehav. Rev. 51 , 108–117 (2015).
Vuust, P. & Kringelbach, M. L. Music improvisation: a challenge for empirical research. in Routledge Companion to Music Cognition (Routledge, 2017).
Norgaard, M. Descriptions of improvisational thinking by artist-level jazz musicians. J. Res. Music. Educ. 59 , 109–127 (2011).
Kringelbach, M. L. & Deco, G. Brain states and transitions: insights from computational neuroscience. Cell Rep. 32 , 108128 (2020).
Deco, G. & Kringelbach, M. L. Hierarchy of information processing in the brain: a novel ‘intrinsic ignition’ framework. Neuron 94 , 961–968 (2017).
Pinho, A. L., de Manzano, O., Fransson, P., Eriksson, H. & Ullen, F. Connecting to create: expertise in musical improvisation is associated with increased functional connectivity between premotor and prefrontal areas. J. Neurosci. 34 , 6156–6163 (2014).
Pinho, A. L., Ullen, F., Castelo-Branco, M., Fransson, P. & de Manzano, O. Addressing a paradox: dual strategies for creative performance in introspective and extrospective networks. Cereb. Cortex 26 , 3052–3063 (2016).
de Manzano, O. & Ullen, F. Activation and connectivity patterns of the presupplementary and dorsal premotor areas during free improvisation of melodies and rhythms. Neuroimage 63 , 272–280 (2012).
Beaty, R. E. et al. Robust prediction of individual creative ability from brain functional connectivity. Proc. Natl Acad. Sci. USA 115 , 1087–1092 (2018).
Daikoku, T. Entropy, uncertainty, and the depth of implicit knowledge on musical creativity: computational study of improvisation in melody and rhythm. Front. Comput. Neurosci. 12 , 97 (2018).
Belden, A. et al. Improvising at rest: differentiating jazz and classical music training with resting state functional connectivity. Neuroimage 207 , 116384 (2020).
Arkin, C., Przysinda, E., Pfeifer, C. W., Zeng, T. & Loui, P. Gray matter correlates of creativity in musical improvisation. Front. Hum. Neurosci. 13 , 169 (2019).
Bashwiner, D. M., Wertz, C. J., Flores, R. A. & Jung, R. E. Musical creativity “revealed” in brain structure: interplay between motor, default mode, and limbic networks. Sci. Rep. 6 , 20482 (2016).
Przysinda, E., Zeng, T., Maves, K., Arkin, C. & Loui, P. Jazz musicians reveal role of expectancy in human creativity. Brain Cogn. 119 , 45–53 (2017).
Large, E. W., Kim, J. C., Flaig, N. K., Bharucha, J. J. & Krumhansl, C. L. A neurodynamic account of musical tonality. Music. Percept. 33 , 319–331 (2016).
Large, E. W. & Palmer, C. Perceiving temporal regularity in music. Cogn. Sci. 26 , 1–37 (2002). This article proposes an oscillator-based approach for the perception of temporal regularity in music .
Cannon, J. J. & Patel, A. D. How beat perception co-opts motor neurophysiology. Trends Cogn. Sci. 25 , 137–150 (2020). The authors propose that cyclic time-keeping activity in the supplementary motor area, termed ‘proto-actions’, is organized by the dorsal striatum to support hierarchical metrical structures .
Keller, P. E., Novembre, G. & Loehr, J. Musical ensemble performance: representing self, other and joint action outcomes. in Shared Representations: Sensorimotor Foundations of Social Life Cambridge Social Neuroscience (eds Cross, E. S. & Obhi, S. S.) 280-310 (Cambridge Univ. Press, 2016).
Rao, R. P. & Ballard, D. H. Predictive coding in the visual cortex: a functional interpretation of some extra-classical receptive-field effects. Nat. Neurosci. 2 , 79–87 (1999).
Clark, A. Whatever next? Predictive brains, situated agents, and the future of cognitive science. Behav. Brain Sci. 36 , 181–204 (2013).
Kahl, R. Selected Writings of Hermann Helmholtz (Wesleyan Univ. Press, 1878).
Gregory, R. L. Perceptions as hypotheses. Philos. Trans. R. Soc. Lond. B Biol. Sci. 290 , 181–197 (1980).
Gibson, J. J. The Ecological Approach to Visual Perception (Houghton Mifflin, 1979).
Fuster, J. The Prefrontal Cortex Anatomy, Physiology and Neuropsychology of the Frontal Lobe (Lippincott-Raven, 1997).
Neisser, U. Cognition and Reality: Principles and Implications of Cognitive Psychology (W H Freeman/Times Books/ Henry Holt & Co, 1976).
Arbib, M. A. & Hesse, M. B. The Construction of Reality (Cambridge Univ. Press, 1986).
Cisek, P. & Kalaska, J. F. Neural mechanisms for interacting with a world full of action choices. Annu. Rev. Neurosci. 33 , 269–298 (2010).
Isomura, T., Parr, T. & Friston, K. Bayesian filtering with multiple internal models: toward a theory of social intelligence. Neural Comput. 31 , 2390–2431 (2019).
Friston, K. & Frith, C. A duet for one. Conscious. Cogn. 36 , 390–405 (2015).
Hunt, B. R., Ott, E. & Yorke, J. A. Differentiable generalized synchronization of chaos. Phys. Rev. E 55 , 4029–4034 (1997).
Ghazanfar, A. A. & Takahashi, D. Y. The evolution of speech: vision, rhythm, cooperation. Trends Cogn. Sci. 18 , 543–553 (2014).
Wilson, M. & Wilson, T. P. An oscillator model of the timing of turn-taking. Psychon. Bull. Rev. 12 , 957–968 (2005).
Download references
Acknowledgements
Funding was provided by The Danish National Research Foundation (DNRF117). The authors thank E. Altenmüller and D. Huron for comments on early versions of the manuscript.
Author information
Authors and affiliations.
Center for Music in the Brain, Aarhus University and The Royal Academy of Music (Det Jyske Musikkonservatorium), Aarhus, Denmark
Peter Vuust, Ole A. Heggli & Morten L. Kringelbach
Wellcome Centre for Human Neuroimaging, University College London, London, UK
Karl J. Friston
Department of Psychiatry, University of Oxford, Oxford, UK
Morten L. Kringelbach
Centre for Eudaimonia and Human Flourishing, Linacre College, University of Oxford, Oxford, UK
You can also search for this author in PubMed Google Scholar
Contributions
The authors contributed equally to all aspects of this article.
Corresponding author
Correspondence to Peter Vuust .
Ethics declarations
Competing interests.
The authors declare no competing interests.
Peer review
Peer review information.
Nature Reviews Neuroscience thanks D. Sammler and the other, anonymous, reviewer(s) for their contribution to the peer review of this work.
Additional information
Publisher’s note.
Springer Nature remains neutral with regard to jurisdictional claims in published maps and institutional affiliations.
Patterns of pitched sounds unfolding over time, in accordance with cultural conventions and constraints.
The combination of multiple, simultaneously pitched sounds to form a chord, and subsequent chord progressions, a fundamental building block of Western music. The rules of harmony are the hierarchically organized expectations for chord progressions.
The structured arrangement of successive sound events over time, a primary parameter of musical structure. Rhythm perception is based on the perception of duration and grouping of these events and can be achieved even if sounds are not discrete, such as amplitude-modulated sounds.
Mathematically, the expected values or means of random variables.
The ability to extract statistical regularities from the world to learn about the environment.
In Western music, the organization of melody and harmony in a hierarchy of relations, often pointing towards a referential pitch (the tonal centre or the tonic).
A predictive framework governing the interpretation of regularly recurring patterns and accents in rhythm.
The output of a model generating outcomes from their causes. In predictive coding, the prediction is generated from expected states of the world and compared with observed outcomes to form a prediction error.
The subjective experience accompanying a strong expectation that a particular event will occur.
An enactive generalization of predictive coding that casts both action and perception as minimizing surprise or prediction error (active inference is considered a corollary of the free-energy principle).
A quantity used in predictive coding to denote the difference between an observation or point estimate and its predicted value. Predictive coding uses precision-weighted prediction errors to update expectations that generate predictions.
Expectations of musical events based on prior knowledge of regularities and patterns in musical sequences, such as melodies and chords.
Expectations of specific events or patterns in a familiar musical sequence.
Short-lived expectations that dynamically shift owing to the ongoing musical context, such as when a repeated musical phrase causes the listener to expect similar phrases as the work continues.
The inverse variance or negative entropy of a random variable. It corresponds to a second-order statistic (for example, a second-order moment) of the variable’s probability distribution or density. This can be contrasted with the mean or expectation, which constitutes a first-order statistic (for example, a first-order moment).
(MMN). A component of the auditory event-related potential recorded with electroencephalography or magnetoencephalography related to a change in different sound features such as pitch, timbre, location of the sound source, intensity and rhythm. It peaks approximately 110–250 ms after change onset and is typically recorded while participants’ attention is distracted from the stimulus, usually by watching a silent film or reading a book. The amplitude and latency of the MMN depends on the deviation magnitude, such that larger deviations in the same context yield larger and faster MMN responses.
(fMRI). A neuroimaging technique that images rapid changes in blood oxygenation levels in the brain.
In the realm of contemporary music, a persistently repeated pattern played by the rhythm section (usually drums, percussion, bass, guitar and/or piano). In music psychology, the pleasurable sensation of wanting to move.
The perceptual correlate of periodicity in sounds that allows their ordering on a frequency-related musical scale.
Also known as tone colour or tone quality, the perceived sound quality of a sound, including its spectral composition and its additional noise characteristics.
The pitch class containing all pitches separated by an integer number of octaves. Humans perceive a similarity between notes having the same chroma.
The contextual unexpectedness or surprise associated with an event.
In the Shannon sense, the expected surprise or information content (self-information). In other words, it is the uncertainty or unpredictability of a random variable (for example, an event in the future).
(MEG). A neuroimaging technique that measures the magnetic fields produced by naturally occurring electrical activity in the brain.
A very small electrical voltage generated in the brain structures in response to specific events or stimuli.
Psychologically, consonance is when two or more notes sound together with an absence of perceived roughness. Dissonance is the antonym of consonance. Western listeners consider intervals produced by frequency ratios such as 1:2 (octave), 3:2 (fifth) or 4:3 (fourth) as consonant. Dissonances are intervals produced by frequency ratios formed from numbers greater than 4.
Stereotypical patterns consisting of two or more chords that conclude a phrase, section or piece of music. They are often used to establish a sense of tonality.
(EEG). An electrophysiological method that measures electrical activity of the brain.
A method of analysing steady-state evoked potentials arising from stimulation or aspects of stimulation repeated at a fixed rate. An example of frequency tagging analysis is shown in Fig. 1c .
A shift of rhythmic emphasis from metrically strong accents to weak accents, a characteristic of multiple musical genres, such as funk, jazz and hip hop.
In Aristotelian ethics, refers to a life well lived or human flourishing, and in affective neuroscience, it is often used to describe meaningful pleasure.
Rights and permissions
Springer Nature or its licensor holds exclusive rights to this article under a publishing agreement with the author(s) or other rightsholder(s); author self-archiving of the accepted manuscript version of this article is solely governed by the terms of such publishing agreement and applicable law.
Reprints and permissions
About this article
Cite this article.
Vuust, P., Heggli, O.A., Friston, K.J. et al. Music in the brain. Nat Rev Neurosci 23 , 287–305 (2022). https://doi.org/10.1038/s41583-022-00578-5
Download citation
Accepted : 22 February 2022
Published : 29 March 2022
Issue Date : May 2022
DOI : https://doi.org/10.1038/s41583-022-00578-5
Share this article
Anyone you share the following link with will be able to read this content:
Sorry, a shareable link is not currently available for this article.
Provided by the Springer Nature SharedIt content-sharing initiative
This article is cited by
Improved emotion differentiation under reduced acoustic variability of speech in autism.
- Mathilde Marie Duville
- Luz María Alonso-Valerdi
- David I. Ibarra-Zarate
BMC Medicine (2024)
Exploring the neural underpinnings of chord prediction uncertainty: an electroencephalography (EEG) study
- Kentaro Ono
- Ryohei Mizuochi
- Shigeto Ymawaki
Scientific Reports (2024)
Effekte von klassischer Musik oder von Heavy Metal bei Mensch und Tier: Implikationen für die Intensivmedizin
- Hans-Joachim Trappe
- Eva-Maria Völkel
- Gerald Reiner
Medizinische Klinik - Intensivmedizin und Notfallmedizin (2024)
Frontal and cerebellar contributions to pitch and rhythm processing: a TMS study
- Silvia Picazio
- Barbara Magnani
- Laura Petrosini
Brain Structure and Function (2024)
Care intervention on psychological outcomes among patients admitted to intensive care unit: an umbrella review of systematic reviews and meta-analyses
- Yafang Zheng
- Lijuan Zhang
- Jingxia Zheng
Systematic Reviews (2023)
Quick links
- Explore articles by subject
- Guide to authors
- Editorial policies
Sign up for the Nature Briefing newsletter — what matters in science, free to your inbox daily.

Music Effects on the Brain
The study of how music affects the memory, motivation, and confidence has been the theme of interest for many researchers in this field. The interrelation of music to the mental and physical health of individuals has been the subject of debate aimed at improving scientific research. Many studies by Cardena (2011, p. 143) have maintained that music has positive effect on our memory, motivation, and confidence.
Music, as Thiam (2006, p. 97) notes, has the power of healing our frequent ailments as human beings. In addition, research validates that the classical forms of music has had an impact on individuals, reporting impressive results on the power of healing particularly ( Effects of Music on the Mind and Brain 2014). Music has a calming effect on the human mind, and an inspiring zeal on the general composure of the body.
Besides, music is an exciting element that hastens human recovery from health illnesses, given its ability to inspire motivation to feel better. More than that, music helps in fighting nervousness, especially due to the comforting impact it has on the mind, the muscles, and the body. Music helps to reduce the impacts of depression.
For instance, when individuals are under depression are gloomy or feeling inadequate, music can offer soothing effects that can raise their composure to normal levels ( Effects of Music on the Mind and Brain 2014).
Dejection has the capacity to moderate the actions of the brain, inhibits the ability of brain to think consistently, and perform certain responsibilities. In this aspect, music induces in individuals an aura of strong will that reverses the impact of depression and autisms in the body (Volkmar 2013, p. 48).
Deficiency of neurotransmitter and serotonin in the brain, according to Koelsch (2014, p. 172), may result in depression. However, listening to music has the ability to inspire the hormones and raise the levels of these elements to equilibrium, making the brain to work optimally.
Soothing musical sounds inspires the production of serotonin levels in the brain, thereby helping in the alleviation of mental depression (Larson 2010). Soothing music has a natural way of making the brain relaxed, creating an aura of confidence in individuals, as well as motivating people in very effective ways.
Among the tried, tested, and trusted benefits of listening to music includes a broad spectrum of ideologies and practicalities that research has sought to validate. To begin with, music has the capacity to relieve anxiety and make the brain more at ease with the body and the environment (Cardena and Winkelman 2011, p. 120).
Anxiety has a unique way of making individuals to degenerate to the lowest ebb of their lives given that it inspires a feeling of fear of the unknown to weigh the body and brain down ( Effects of Music on the Mind and the Brain 2014). The outcome of the unknown makes individuals worried, and this might lead to temporary brain malfunction with probable greater consequences in the event that this trend escalates.
Increased levels of anxiety, according to Hallam, Cross, and Thaut (2009, p. 62), may lead to stress, and, further, culminate into insomnia. Additionally, lengthy instances of worry may lead to ailments related to nervousness. Though if noted in time, making such individuals to listen to music can be instrumental in checking the menace (Koelsch 2014, p. 175).
Introducing music to individuals undergoing extreme levels of anxiety can help in relaxing their brain and raise their hormones back to normal levels ( Effects of Music on the Mind and the Brain 2014).
Used in this way, music can help in calming the body nerves and, finally, sooth the mind to easiness. Flat musical notes can also be instrumental in inducing sleep in individuals with sleep-related disorders, thereby helping in their brain development.
Music has the capacity to motivate individuals as it affects both the learning and the thinking processes (Jensen 2005, p. 309).Studies in sciences suggest that soft background music stimulates the mind and the brain to absorb knowledge and retain data ( Effects of Music on the Mind and the Brain 2014). Individuals listening to soothing beats while doing some work help them to work more rapidly.
In the process, they become more effective too. Music used in this way, therefore, helps individuals to feel motivated, and people become more positive about work in general. According to Cardena and Winkelman (2011, p. 127), research in this area indicates that music makes individuals more consistent in learning as it brings about remarkable progresses in fast tracking motivation in individuals.
Students can also find meaningful motivation in their respective studies while listening to music given that music breaks the monotony of everyday classroom work. As Hallam et al. (2009, p. 65) note, students who attend classes that prescribe certain kinds of musical notes while studying in the laboratory record greater progress results as they become more involved in their tasks than those with no musical notes.
Listening to some pleasant music, while doing some boring work or difficult task will eventually spur motivation to make the work look easier (Curtis 2008). Normally, an individual working while listening to some soft music at the background usually records least interruptions from other environmental hitches.
Due to least interruption, such people concentrate more on what they do, thus making them produce quality results in their tasks.
Research in this area also holds that music has the capacity to boost confidence, especially in individuals with low self-esteem (Hallam et al. 2009, p. 72). Music has an affirmative influence in developing the interactive skills of entities. Lack of sureness as well as no aspiration to comprehend is one of the attributes to letdown.
Accordingly, it is not always the inability of people to learn, rather, it is due to lack of confidence in what one does (Avanzini 2003, p. 296). Individuals have every endowment to learn given motivation and chance to do so.
Indeed, it is only that the prevailing situations in different settings may make some people to own up and let their low self-esteem weigh them down. Learners attaining poor grades in learning institutions, for example, are not essentially devoid of the required intellect.
Most educators agree that musical involvement advances students’ self-discipline, coordination, dexterity, thinking skills, self-esteem, creative abilities, listening skills, and personal expression, each of which supports learning in very profound ways. Most music educators, though, are not aware of specific research that will support such feelings and observations ( Music and Student Development 2014).
As Thiam (2006, p. 117) notes, the students’ disinterest in the subjects in question leads them to record poor grades. However, music classes can help the learners to regain composure and fight out their low-esteem lag that makes them perform dismally. Music, therefore, helps in boosting learner confidence, encouraging them to explore new ideas.
In so doing, they venture in new fields giving them the basic orientation of people, places, and ideas that they previously felt was impermeable. From this perspective, music increases people’s ability in believing in themselves and in increasing their capacity to think big.
The effects of music in the recovery of physiological difficulties experienced after a stressful aperture induced by aversive visual stimuli are equally great.
Studies show that the relaxing nature of music is effective in regulating the spectral of the frontal temporal lobe, rejuvenating the skin conductance, moderating the heart beat variability, creating the respiration ambience, as well as encrypting the facial capillary blood current (Cardena and Winkelman 2011, p. 128).
Under normal circumstances, aversive visual stimulation evokes heart rate deceleration and decrease facial blood flow that could be detrimental in causing visual impairment. In such situations, respiration rate gets to unguided levels that may make the brain not to function optimally.
Studies show that pleasant music has the capacity to restore the baseline levels of several parameters and the recovery process of most of the body tissues (Sokhadze 2007, p. 37). Music relaxes the body thereby exerting positive modulatory effects on respiratory and cardiovascular activity.
Such activities regulate increased heart rate with the ability to balance the heart period variability, while regulating vascular blood flow, as well as respiration rate in the course of post-stress recovery (Sokhadze 2007, p. 43). In much of the research done in this area, data has been consistent to the effect that positive emotions guaranteed by music facilitate the process of neurochemistry recovery that arise from negative emotions
Invariably, music stands out as a universal feature in human development, partly due to its power and capacity to evoke strong sensations and influence moods. Investigation both in the scopes of science and art continue to develop their expanse of the richness of music to the wellbeing of man (Cardena and Winkelman 2011, p. 131).
In numerous studies, the neural correlation of music with its capacity to evoke emotions in individuals continues to be an invaluable element in the understanding and study of human emotions (Koelsch 2014, p. 177).
It is no doubt that functional neuroimaging research based on music and feeling demonstrate that music has the capacity to moderate actions in the mind much of which are critically encompassed in developing emotions.
Several brain structures such as hypothalamus, hippocampus, cingulate cortex insula, orbitofrontal cortex, amygdala, and nucleus acumens heavily rely on hormones to inspire them to perform their desired functions (Cardena and Winkelman 2011, p. 139).
The possibilities of music to inspire and control hormones to act in these arrangements have imperative inferences for the application of music in the management of neurological and psychiatric ailments.
Essentially, emotions inspire the brain and enhance the processes of memory (Norden, Reay and Leven 2007, p. 60). Since music has the ability to evoke strong emotions, it could be instrumental in inspiring the brain to rise to the occasion and perform its duties optimally. To that effect, music arouses individuals either about a particular music, an event, or about an episode or information related to a particular music.
Neuroscience studies enhance insights into the role of music in relaxing the brain to inspire memory. Playing musical instruments, according to Jensen (2005, p. 313), acts as a multi-sensory as well as a motor experience generating emotions and motion tapping of the finger to dancing. Seen in this way, music engages the pleasure while acting as the reward element in the brain systems (Norden et al. 2007, p. 61).
Moreover, music has the potential to alter brain function as well as the brain structure when done well for over a period. Accordingly, intense musical tutelage and exposure has the capacity to generate and inspire new processes in the brain, and, normally, this occurs at different stages in life (Jensen 2005, p. 314).
As Norden et al. 2007, p. 68) observe, music is instrumental in developing the brain with a range of impacts based on creativity, the learning process, and cognition among other things.
Whether the purpose of music has to be for recreation, entertainment, or enhancement of moods, Koelsch (2014, p. 178) supports Forinash (2001, p. 33) argument that many people listen to music for various reasons, especially for its inspiring value.
Because of its potent and ubiquity, music is very effective in the construction and correction of autobiographical memories while helping in building judgments about others and on oneself (Forinash 2001, p. 34). Several elements in human development factor in the role of music such as aiding in memory formation and recalling of autobiographical facets and episodic information.
From the forgoing analysis, it becomes clear that music has a vital role in developing individuals in very many special ways. In essence, music is an essential and extremely valuable tool in the way individuals learn. Music has a calming effect on individuals and, indeed, an inspiring zeal on the composure of individuals generally.
Music has the ability to incite hormones exciting and speed up human recovery from health ailments given its ability to inspire motivations ( Music and Student Development 2014). Apart from that, music’s comforting influence aids in fighting nervousness since it relaxes the muscles, the brain, and eventually the whole body.
Neuroscience, in particular, offers firsthand and profound perceptions into the function of music in composure of sentiments. Moreover, neuroscience studies enhance understandings into the role of songs in feelings, relaxing the brain to inspire retention. Moreover, music helps to reduce the impacts of depression; it is true that when individuals undergo depression, they become gloomy, making them feel inadequate.
Music, therefore, can offer the basic soothing effects that can bring the discomfits back to normal levels. As observed, depression has the capacity to moderate brain functions, inhibits the ability of the brain to think and reason with a high level of consistency, and carry out certain tasks. When played along, it induces in individuals an aura of strong will that reverses the impact of depression weighing down the body.
Finally, since deficiency of neurotransmitter and serotonin in the brain may go down because of depression, music offers itself as a correctional factor that incites the hormones needed to raise neurotransmitter and serotonin to normal levels. In retrospect, listening to music has the ability to inspire the hormones and raise the levels of the brain structures to equilibrium, making the brain to work optimally.
In conclusion, as the case may be, comforting musical sounds inspires the production of serotonin levels, thereby helping in the alleviation of mental dejection. Soothing music, therefore, has a natural way of making the brain relaxed, creating an aura of confidence in individuals, while motivating to rise to the occasion and feel better.
Avanzini, G 2003, The neurosciences and music , New York Academy of Sciences, New York.
Cardena, E 2011, Altering consciousness multidisciplinary perspectives , Praeger, Santa Barbara, California.
Cardena, E., and Winkelman, M 2011, Altering Consciousness, 2 Volumes Multidisciplinary Perspectives , ABC-CLIO, Santa Barbara.
Curtis, B. W 2008, Music makes the nation nationalist composers and nation building in nineteenth-century Europe Cambria Press, Amherst.
Effects of Music on the Mind and the Brain 2014. Web.
Forinash, M 2001, Music therapy supervision , Gilsum, NH, Barcelona.
Hallam, S., Cross, I., and Thaut, M. H 2009, The Oxford handbook of music psychology , Oxford University Press, Oxford.
Jensen, E 2005, Teaching with the brain in mind (2nd ed.), Association for Supervision and Curriculum Development, Alexandria.
Koelsch, S 2014, ‘Brain correlates of music-evoked emotions’, Nature Reviews Neuroscience, vol. 15, no. 17, pp. 170–180.
Larson, D 2010, The effects of chamber music experience on music performance achievement, motivation, and attitudes among high school band students, Cambridge University Press, Cambridge.
Music and Student Development 2014. Web.
Norden, J., Reay, A., and Leven, J 2007, Understanding the brain , Teaching Co., Chantilly, VA.
Sokhadze, E 2007, ‘Effects of Music on the Recovery of Autonomic and Electrocortical Activity After Stress Induced by Aversive Visual Stimuli’, Applied Psychophysiology and Biofeedback , vol. 32, no. 1, pp. 31-50.
Thiam, P. B 2006, Effects of school band experience on the motivation of high school students , Springer, New York.
Volkmar, F. R 2013, Encyclopedia of autism spectrum disorders , Springer, New York.
- Chicago (A-D)
- Chicago (N-B)
IvyPanda. (2023, November 22). Music Effects on the Brain. https://ivypanda.com/essays/music-and-neuroscience/
"Music Effects on the Brain." IvyPanda , 22 Nov. 2023, ivypanda.com/essays/music-and-neuroscience/.
IvyPanda . (2023) 'Music Effects on the Brain'. 22 November.
IvyPanda . 2023. "Music Effects on the Brain." November 22, 2023. https://ivypanda.com/essays/music-and-neuroscience/.
1. IvyPanda . "Music Effects on the Brain." November 22, 2023. https://ivypanda.com/essays/music-and-neuroscience/.
Bibliography
IvyPanda . "Music Effects on the Brain." November 22, 2023. https://ivypanda.com/essays/music-and-neuroscience/.
- How Serotonin Affects the Brain and Addictions
- Shelby Steel interview with Bill Moyers
- Serotonin Syndrome Article by Buckley
- Compare GUDEA with Amenemhat II
- Neurotransmitter: Aspects of the Serotonin
- Gibb’s Reflective Cycle: COVID-19 Scenario
- DHL & Qantas Flight 32: Teamwork and Creative Approaches
- How Technology Is Changing Our Minds
- Conflict in ‘The Most Dangerous Game’ by Connell
- The Serotonin Theory of Depression by Moncrieff et al.
- Schwarzkopf' Leadership Style and Success as a Military General
- Depression Diagnosis and Theoretical Models
- Marci: Analysis of a Counseling Situation
- Depression and Workplace Violence
- Case Conceptualization of Tricia Davis
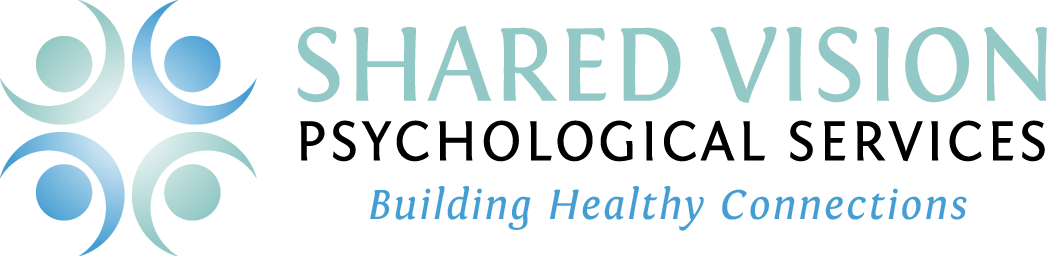
An Introduction to How Music Affects Your Brain
Author: Dr. Pamela McMullin
As a psychologist and a musician, I remember my mother telling me to practice my violin when I was young. When I complained – which I did, often- she would shout up the stairs that it was good for me . As it turns out, she was right. Although she was likely unaware of exactly how or why it was good for me, research now shows us that listening to, and especially playing music, exercises almost every part of the brain.

Contrary to the notion that art and music are processed by the right hemisphere of the brain, and language and mathematics by the left, Daniel J. Levitin reports in his New York Times Bestseller, “This is Your Brain on Music”, that music is processed throughout the brain. Listening to music, playing and writing music engages nearly every area of the brain that researchers have identified. How do we know this? Partly through brain scans; specifically, Functional MRIs and PET scans have given neuroscientists the ability to see how the brain works in real time. Using base line scans, then having patients listen to music, researchers are able to see multiple areas of the brain light up as the patient processes sound.
The following are a just a sample of the amazing ways music affects the brain:
1) Music can strengthen our bonds with others.
A study by Florida International University’s Community Based Research Institute has found that ensemble based musical instruction for young children (ages 8-17) had significant increases on kid’s competence, confidence, caring, character and connection.
2) Music reduces stress and depression.
Advertisers and filmmakers know the power music has to evoke emotion. Studies have shown that listening to and playing music helped reduce pain and anxiety associated with cancer treatments. It is long known that listening to music helps people stick to an exercise routine, train for a physical competition, heal from a break up, undergo a dental procedure, or soothe an upset infant.
3) Want to relieve anxiety? Join a choir.
Beyond the sense of togetherness one can feel as being part of a choir, researchers have studied the benefits that choral singing can have on our heart rate. Breathing and heart rates are directly affected by melody, and are shown to slow or quicken with the tempo. Studies have even shown that individuals’ breathing and heart rates in a choir actually slowed in unison. Overall, singing requires a slower than normal respiration rate, and as relaxation techniques often involve deep breathing, this makes sense. By the way, study results weren’t dependent on whether or not you consider yourself a good singer! Just singing in a choir- even a mediocre one- can give you similar benefit.
4) While it may not make us smarter, music does enhance arousal and performance as it positively affects how we feel.
Dopamine is a neurotransmitter associated with pleasure. It carries information between neurons in the brain and helps in the regulation of attention, movement, learning and emotions—known specifically to contribute to feelings of pleasure we experience during eating, sleeping, and sex. We now know that listening to music also causes the release of dopamine -and this isn’t specific to a particular type of music. Patients who were exposed to PET scans while listening to a symphony had the same dopamine response as those exposed to other types of music. Daniel Abrams, author of this study and researcher, found that when we listen to music, our brains are doing much more than simply processing sound. Music activated all areas of the brain associated with planning, attention, movement, and memory as dopamine was released.

5) Your musical preferences may be related to the music your parents were playing.
We know that the auditory system develops in the fetus around 16 weeks of pregnancy- meaning inside the womb, the baby is hearing the sounds of their world. Using a research technique called The Conditioned Head Turn procedure, studies have looked at how playing music during pregnancy can affect the baby’s development and preferences. Specifically, they showed that the babies studied remembered and even preferred the music played to them in the womb when measured a year after birth. The take-away from this is that prenatal brains are able to encode memories of music and retrieve them over time- long before language and awareness.
6) Ever wonder why you can’t get that song out of your head?
That is actually a not uncommon phenomenon psychologists refer to as involuntary musical imagery (INMI), or “earworms”. It might have something to do with the music or song, itself. Looking at the top 100 songs chosen by the 3000 people studied who experienced having earworms, researchers identified some common elements of the melodies. Songs most likely to get stuck had more upbeat tempos, an unusual interval structure, or chord or chorus repetitions. An example of the top “earworms” people find most difficult to get out of their heads: Bohemian Rhapsody- Queen, YMCA- Village People, and Happy- Pharrell Williams.

There is much more to learn about how music affects your brain. If you’re interested in learning more about the neuroscience of music, how we get certain jingles stuck in our heads, or why 10,000 hours, not talent, makes virtuosos- read Daniel Levitin’s book. For more information on how playing an instrument can affect the brain, check out the Ted Talk below! If you’d like to strengthen your brain functioning in all the ways summarized above, turn up the music!
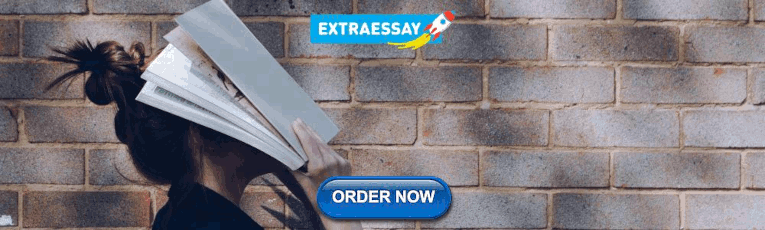
References: https://www.inc/john-rampton/the-benefits-of-playing-music-help-your-brain-more.html
Levitin, daniel j. 2006. this is your brain on music: the science of a human obsession. usa: penguin., https://musiceducationworks.com/2016/06/19/a-childs-brain, https://www.psychologytoday.com/us/blog/your-musical-self/201101/why-listening-music-makes-us-feel-good, https://stempel.fiu.edu/cbri-researchers-find-after-school-ensemble-music-programs-positively-affect-students-character-competence-and-caring/, https://www.ted.com/talks/anita_collins_how_playing_an_instrument_benefits_your_brainlanguage=en, university of california: http://dornsife.usc.edu/bci/brain-and-music/, vickhoff, b., malmgren, h., åström, r., nyberg, g. f., ekström, s., engwall, m., . . . jörnsten, r. (2013). erratum: music structure determines heart rate variability of singers. frontiers in psychology, 4. doi:10.3389/fpsyg.2013.00599, welch, ashley., 2016, cbs news. psychologists identify why certain songs get stuck in your head..
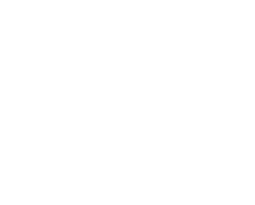
- FAQs & COVID-19 Info
- Intake Forms
- Child Therapy
- Adolescent Therapy
- Adult Therapy
- Couples Therapy
- Family Therapy
- Parent Consultation
- Circle of Security™
- Events & Webinars
- Community Connections
- Organizations
- Professional Training & Consultation
- Advanced Doctoral Externship
- APA Accredited Doctoral Internship
- Postdoctoral Fellowship
- Client Portal
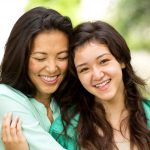
Collins Memorial Library
Music 222: music of the world's peoples.
- Getting Started
- Research Questions
- Developing a Thesis
- Writing & Citing
Argumentative Paper Thesis
- Proposed answer to a research question
- Should make a claim and argue it
- Thesis = Topic + a claim (attitude or opinion) + major points (specifics about the points you will use to explain your claim)
- A good thesis has a definable, debatable claim
- Your thesis statement should be specific—it should cover only what you will discuss in your paper and should be supported with specific evidence.
- Your topic may change as you write, so you may need to revise your thesis statement to reflect exactly what you have discussed in the paper.
How to Write a Thesis Statement
A thesis statement is not a statement of fact. It is an assertive statement that states your claims and that you can prove with evidence. It should be the product of research and your own critical thinking. There are different ways and different approaches to write a thesis statement. Here are some steps you can try to create a thesis statement:
1. Start out with the main topic and focus of your essay.
Example: youth gangs + prevention and intervention programs
2. Make a claim or argument in one sentence.
Example: Prevention and intervention programs can stop youth gang activities.
3. Revise the sentence by using specific terms.
Example: Early prevention programs in schools are the most effective way to prevent youth gang involvement.
4. Further revise the sentence to cover the scope of your essay and make a strong statement.
Example: Among various prevention and intervention efforts that have been made to deal with the rapid growth of youth gangs, early school-based prevention programs are the most effective way to prevent youth gang involvement.
Thesis Examples from Published Research
Take a look at the following articles and identify the thesis statement. Why is it an effective or not effective thesis?
1. White, Theresa Renee. “Missy ‘Misdemeanor’ Elliott and Nicki Minaj: Fashionistin' Black Female Sexuality in Hip-Hop Culture—Girl Power or Overpowered?” Journal of Black Studies , vol. 44, no. 6, 2013, pp. 607–626. JSTOR , http://ezproxy.ups.edu/login?url=https://www.jstor.org/stable/24572858 . Accessed 30 Nov. 2020.
2. McNally, James. "Azealia Banks's "212": Black Female Identity and the White Gaze in Contemporary Hip-Hop." Journal of the Society for American Music 10.1 (2016): 54-81. ProQuest, http://ezproxy.ups.edu/login?url=https://www-proquest-com.ezproxy.ups.edu:2443/docview/1882381971?accountid=1627 . Accessed 30 Nov. 2020.
Thesis Statement Tutorial
Good Thesis Tips
- Ensure your thesis is provable. Do not come up with your thesis and then look it up later. The thesis is the end point of your research, not the beginning. You need to use a thesis you can actually back up with evidence.
- First, analyze your primary sources . Look for tension, interest, ambiguity, controversy, and/or complication. Ask questions about the sources.
- Anticipate the counterarguments. Every argument has a counterargument; if yours doesn't, it's not an argument (may be a fact or an opinion). If there are too many arguments against it, find another thesis.
- Communicate a single, overarching point rather than multiple points that may be too difficult or broad to support
Examples of Non-Debatable and Debatable Thesis Statements
Example of a non-debatable thesis statement:
Pollution is bad for the environment.
Example of a debatable thesis statement:
At least 25 percent of the federal budget should be spent on limiting pollution.
The North and South fought the Civil War for many reasons, some of which were the same and some different.
While both Northerners and Southerners believed they fought against tyranny and oppression, Northerners focused on the oppression of slaves while Southerners defended their own right to self-government.
- << Previous: Research Questions
- Next: Books >>
- Last Updated: Mar 15, 2024 11:06 AM
- URL: https://library.pugetsound.edu/music222
- Election 2024
- Entertainment
- Newsletters
- Photography
- Personal Finance
- AP Investigations
- AP Buyline Personal Finance
- Press Releases
- Israel-Hamas War
- Russia-Ukraine War
- Global elections
- Asia Pacific
- Latin America
- Middle East
- Election Results
- Delegate Tracker
- AP & Elections
- March Madness
- AP Top 25 Poll
- Movie reviews
- Book reviews
- Personal finance
- Financial Markets
- Business Highlights
- Financial wellness
- Artificial Intelligence
- Social Media
Maggie Rogers on ‘Don’t Forget Me,’ the album she wrote for a Sunday drive
Maggie Rogers wrote and recorded her new album, “Don’t Forget Me,” over five days last winter. The songs came quickly and chronologically, as if she was writing “different scenes in a movie.” (April 12)
Maggie Rogers performs on Day 3 of the Lollapalooza Music Festival on Saturday, Aug. 5, 2023, at Grant Park in Chicago. (Photo by Amy Harris/Invision/AP)
- Copy Link copied
NEW YORK (AP) — Maggie Rogers wrote and recorded her new album, “Don’t Forget Me,” over five days last winter. The songs came quickly and chronologically, as if she was writing “different scenes in a movie.”
“I’ve been writing songs now for 15 years, and so I think I’ve just come into this place where I really trust my process and my craft. I think because of that, I was in a place where I was willing to just play,” Rogers said in a Zoom interview ahead of the album’s release.
The result is a record that Rogers calls “relaxed,” one that finds her “unguarded and present.”
The mood is lighter than Rogers’ electric 2022 album “Surrender,” which was in many ways a release of pent-up pandemic energy — a collection of songs that begged to be experienced live and with a crowd. And it leans more heavily on acoustic sounds than 2019’s “Heard it in a Past Life,” the first album Rogers released after a video of Pharrell Williams reacting to her song, “Alaska,” in a New York University class went viral.
As Rogers explains it : If “Heard it in a Past Life” is air and “Surrender” is fire, “Don’t Forget Me” is earth.
Like her past projects — and her studies at Harvard’s Divinity School — community is a through-line of “Don’t Forget Me.” Rogers name-drops friends and tells their stories alongside hers. She has welcomed the way the new songs have united crowds, and looks forward to continuing to foster that joyous, present, environment on tour.
“I’m excited to be able to meet people in it,” she said, referencing the album’s Friday release.
That community-first quality of her music is something that fans, too, embrace: New York’s Gaia Music Collective, for example, organized a “one-day choir” of Rogers’ song “Light On.” Four hundred people gathered to learn and perform an arrangement of the song, a cappella.
“She also is thinking about music as a connective force, as a thing that can bring us and our stories together,” said Matt Goldstein, the group’s founder and co-director. “It’s no accident that her music feels good to sing together.”
This interview has been edited for clarity and brevity.
AP: “Surrender” felt like an album that had to be moved through and experienced with a crowd. How do you see this album being embodied?
Rogers: This record was always made for the car. I really wanted to make a record that felt like a Sunday driving record, because to me, those are some of the most intimate moments that I’ve had with music or with an album — when I’m singing along to a song in the car and it feels like that artist or that song is like a friend in my passenger seat. Those are some of my favorite records, and they’re the records I turn to for comfort over and over again. And you know, in this big, crazy, completely insane, existential, world, if that’s something that I could offer to the world through my music, I think that that is really special.
AP: Could you say more about how you see your three studio albums in relation to each other? I liked how you described each of them as elements — air, fire, earth.
Rogers: I think categorizing them in that way is just a way to help give context to them. To me, they’re all really important reflections on different periods in my life. And my songwriting is pretty consistent at the center of all these things. It’s mostly the way that like my producer brain has chosen to dress up the songs at their core, and that has more to do with creative expression or curiosity more than anything.
In each record, I’m just trying to be as present and authentic as I can. “Don’t Forget Me,” what I love about it, is that it’s this really, like kind of woven tapestry. Like in so many ways I created a character that sort of led me through this album, rather than trying to make a snapshot of my life in the exact moment. But there are real truths woven into that character and into those stories.
And you know, it comes out two weeks before I turn 30 and in a way it feels like this big ode to my 20s and everything I’ve experienced. Even if the narrative plot line isn’t exactly 1:1 with my life, the essence of all of the feelings within all of the songs feels really, really truthful.
AP: Looking ahead, what do you think you learned after putting what you had studied at Harvard into practice while touring last year?
Rogers: It’s funny because I spend all this time thinking about live music and the way people come together around it, and I had this thought that when I got on stage that it would be like, you know, that meme of the woman with the math problem above her head? I was like, that’s going to be me. And then I got on stage. And what I love about being on stage is that I’m not thinking, I’m just moving. I’m just feeling. It’s like deeply instinctual.
When I think about the tour that I’ll be on for the next year, I think I’m mostly just really excited to have fun. Like, I’ve worked for so many years and been in so many bands, whether it was like at some underground club in New York City or being 18 and playing in bars or being on the road in a van at the beginning of my career, that now I feel really comfortable on stage and I love playing live. I’m so happy that live music is back in this way.
AP: You posted a video around the holidays about a journal entry you found from the end of your time at NYU.
Rogers: So trippy.
AP: How does that kind of reflective writing fit into your daily practice and songwriting now?
Rogers: It’s such a massive part of my life. I mean, I write every day. I kind of can’t sleep without it. It’s usually the last thing I do at the end of the day. And it, like, really is a meditation.
I wrote a ton through grad school and have continued working on essays and I’m turning my master’s thesis into a book. My long form writing practice feels as much a part of my life as my short form songwriting practice, and it helps me to stay really present in my life because I’m paying attention to detail all the time.
AP: You said that writing this album felt like writing scenes from a movie. Do you look to films for inspiration when you’re developing a storyline and character like this?
Rogers: No, I mean, the movies that I love often have a strong female lead — like, “10 Things I Hate About You” was a really big, big part of “Surrender.” And “Thelma and Louise” was a big part of this record. I don’t know — maybe it makes me a basic b—, but, like, I’m a lover of a Meg Ryan rom-com, or a Julia Roberts movie. But that’s just what I like. That to me has the same sense of comfort as the album that is the passenger in the passenger seat that you’re singing along to in your car — they hold the same space for me.

An official website of the United States government
The .gov means it’s official. Federal government websites often end in .gov or .mil. Before sharing sensitive information, make sure you’re on a federal government site.
The site is secure. The https:// ensures that you are connecting to the official website and that any information you provide is encrypted and transmitted securely.
- Publications
- Account settings
Preview improvements coming to the PMC website in October 2024. Learn More or Try it out now .
- Advanced Search
- Journal List
- v.42(45); 2022 Nov 9
Music and Brain Circuitry: Strategies for Strengthening Evidence-Based Research for Music-Based Interventions
Wen grace chen.
1 Division of Extramural Research, National Center for Complementary and Integrative Health, National Institutes of Health, Bethesda, Maryland, 20892
John Rehner Iversen
2 University of California-San Diego, La Jolla, California 92093
Mimi H. Kao
3 Tufts University, Medford, Massachusetts 02155
Psyche Loui
4 Northeastern University, Boston, Massachusetts 02115
Aniruddh Dhiren Patel
Robert j. zatorre.
5 Montreal Neurological Institute, McGill University, Montreal, Quebec H3A2B4, Canada
Emmeline Edwards
Author contributions: E.E. and W.G.C. conceptualized the review; P.L. and W.G.C. constructed the outline of this review; W.G.C., E.E., J.R.I., M.H.K., P.L., A.D.P., and R.J.Z. were involved in literature review and contributed to different sections and editing of the manuscript; W.G.C. contributed to the coordination, integration, and submission of the manuscript.
The neuroscience of music and music-based interventions (MBIs) is a fascinating but challenging research field. While music is a ubiquitous component of every human society, MBIs may encompass listening to music, performing music, music-based movement, undergoing music education and training, or receiving treatment from music therapists. Unraveling the brain circuits activated and influenced by MBIs may help us gain better understanding of the therapeutic and educational values of MBIs by gathering strong research evidence. However, the complexity and variety of MBIs impose unique research challenges. This article reviews the recent endeavor led by the National Institutes of Health to support evidence-based research of MBIs and their impact on health and diseases. It also highlights fundamental challenges and strategies of MBI research with emphases on the utilization of animal models, human brain imaging and stimulation technologies, behavior and motion capturing tools, and computational approaches. It concludes with suggestions of basic requirements when studying MBIs and promising future directions to further strengthen evidence-based research on MBIs in connections with brain circuitry.
SIGNIFICANCE STATEMENT Music and music-based interventions (MBI) engage a wide range of brain circuits and hold promising therapeutic potentials for a variety of health conditions. Comparative studies using animal models have helped in uncovering brain circuit activities involved in rhythm perception, while human imaging, brain stimulation, and motion capture technologies have enabled neural circuit analysis underlying the effects of MBIs on motor, affective/reward, and cognitive function. Combining computational analysis, such as prediction method, with mechanistic studies in animal models and humans may unravel the complexity of MBIs and their effects on health and disease.
Introduction
Music is an integral part of every human society. Music can bring pleasure, calm anxiety, soothe sorrow, inspire and/or stimulate movement, and promote social connections. Musical experiences may also have the remarkable ability to enhance brain and cognitive development, improve function and well-being, optimize the quality of life, and possibly ameliorate the symptoms of a broad range of diseases and disorders.
Recognizing the untapped therapeutic potentials of music-based interventions (MBIs), the National Institutes of Health (NIH), John F. Kennedy Center for the Performing Arts, and National Endowment for the Arts formed a collaborative partnership, Sound Health, in 2016. The journey started with a jointly organized workshop, Music and the Brain: Research Across the Lifespan, which was held in January 2017, to evaluate the state of basic and applied music research. In this meeting, a diverse panel of experts discussed the impact of music on the brain across the lifespan (childhood, adulthood, and aging) and made recommendations for enhancing research in each of these domains ( Cheever et al., 2018 ). In the 2018 Dialogues Between Neuroscience and Society lecture, musician Pat Metheny discussed with a panel of Society of Neuroscience members the impact of music on the brain and the role of music in healing. Soon after, NIH issued a series of special funding opportunities to promote basic, mechanistic, and clinical research on MBI ( Chen et al., 2018 , 2020 ; Riddle et al., 2018a , b , 2020a , b ). In 2021, NIH organized three workshops focusing on Laying the Foundation: Defining the Building Blocks of Music-Based Interventions, Assessing and Measuring Target Engagement—Mechanistic and Clinical Outcome Measures for Brain Disorders of Aging, and Relating Target Engagement to Clinical Benefit—Biomarkers for Brain Disorders of Aging, respectively. Discussions at these workshops resulted in the development of the NIH Music-Based Interventions Toolkit ( Edwards et al., 2022 ). NIH also intends to support the development of research networks on MBIs with a particular emphasis on developing compelling research frameworks; identifying consistent terminology and taxonomy to guide future clinical research; supporting interdisciplinary collaborations and pilot studies to test novel mechanistic hypotheses; and developing strong mechanistic measures, outcomes, and biomarkers, with a special emphasis on several brain diseases and disorders, such as pain, Alzheimer's disease, Parkinson's disease (PD), stroke, and/or aging.
A central thesis involved in all these endeavors is the question of how MBIs achieve their therapeutic potentials. The power of music to influence movement, emotion, learning, and behavior is enormous. One hypothesis is that music's impact is linked to its ability to engage multiple neural systems of the brain. But what is the support for such a conclusion? If we are ever to harness music's multitude of influences, we need a solid understanding of the neural circuitry involved and rigorous evidence about how it is engaged by music. From a brain circuitry perspective, the idea may seem straightforward: as musical sounds are first processed by auditory mechanisms in our CNS, therapeutic effects derived from MBIs would most likely require the engagement of brain circuits and other physiological systems that are directly or indirectly connected to the auditory neural circuitry involved in perceiving and processing elements of music.
To test this idea scientifically, we first need to have clear definitions or characterizations of what music and MBIs are. Basic constituents of music include melody, harmony, and rhythm ( Vuust et al., 2022 ). Each of these three elements has countless sequences, tempos, and dynamics or loudness of sound, and they can be combined in numerous ways. This enormous heterogeneity in musical contents is then implemented on a variable target population of MBIs ( Loui, 2020 ) ( Fig. 1 A ). The mode of MBI delivery also varies ( Fig. 1 B ). The content of music may be heard in a receptive mode ( Hanser, 2016 ), or it may be performed or presented by an individual in an active engagement mode often requiring some degree of motor activities, such as singing, playing an instrument, dancing, or even composing. In addition, MBIs may have a social interaction component if they are delivered in a group setting, such as listening in a concert hall with an audience, performing music as a group, or interactions between the performers and the listeners; or delivered by a music therapist to a patient or a group of patients, for instance. In MBI research, clearly describing the intervention itself, including music content and mode of delivery, in addition to other common intervention parameters, such as duration and frequency, may be the first important step. Music and MBIs have been associated with a variety of brain functions and disorders. Therefore identifying and testing the neural network connections between the auditory neural pathways where the sounds of music are first processed and other brain networks, such as motor, affective/reward, cognitive, as well as other sensory circuits, including pain, vision, and interoception, which impacts other physiological systems ( Chen et al., 2021 ), will be critical to help us understand how MBIs may exert their therapeutic effects ( Loui, 2020 ) ( Fig. 1 C ).

Evidence-based research on MBIs. A , Illustrative examples of components of music, including melody, harmony, and rhythm. B , Examples of the modes of delivery of MBIs. Receptive modes: when a subject passively listens to musical components. Active modes: when a subject actively performs musical components. Solo modes: when a subject is passively listening to (receptively) or actively performing musical components. Group/Social modes: when a subject is receiving or performing music in a group setting, or when a subject is, or subjects are, interacting with a music therapist or therapists. C , Brain circuits engaged in potential therapeutic effects by MBIs. Musical components are first processed through the auditory pathway. Evidence has emerged to support neural network connections between auditory and motor or affective/motivational systems, which may underlie MBI's therapeutic effects on related diseases, such as PD, stroke, stress, anxiety, and addition. The neural network connections between the auditory pathway and cognitive or other sensory systems, such as interoception, somatosensation, nociception, and vision, remain to be explored for implications on diseases, such as Alzheimer's disease, dementia, cardiovascular diseases, and pain. D , Examples of biological/model systems studied in MBI research include birds, rodents, nonhuman primates, and humans. E , Examples of technologies used to study MBIs. Examples of brain imaging technologies include MEG, EEG, and fMRI. An example of brain stimulation technology is TMS. An example of behavior capturing technology is a motion-capture and tracking system.
This review article highlights research findings presented at the 2022 Society of Neuroscience Symposium Music and Brain Circuitry: Strategies for Strengthening Evidence-Based Research . Specifically, we will begin with the complexity of MBIs and the importance of neuroscience approaches to help address fundamentals of interventions, such as dosages. Comparative studies across multiple species, including birds, rodents, nonhuman primates, and humans ( Fig. 1 D ), also offer significant insights into neural circuits involved in MBIs with a high level of rigor, especially regarding perception of music rhythm and the auditory and motor neural systems involved. Multiple brain imaging tools, including EEG and MRI, brain stimulation approaches, such as transcranial magnetic stimulation (TMS), as well as innovative behavioral analysis using technologies, such as motion capture and prediction analysis methods ( Fig. 1 E ), further allowed investigators to probe the neural mechanisms and explore the neural network connections underlying the effects of music and MBIs on a variety of brain function and behavioral disorders.
Fundamentals of music-based interventions
The myriad musical contents conferred by countless combinations of its constituents pose a major challenge for MBIs, especially in defining the intervention and maintaining consistency. In basic and mechanistic research, it may be possible to study musical constituents in a reductionist way by focusing on one or a few specific combinations or forms of constituents. In contrast, more holistic approaches by the music therapy community, for example, seem to share the general consensus that there is no one-size-fits-all program: while self-selected music confers the most therapeutic benefit for a variety of clinical applications, the music therapist consults with clients and caregivers to come up with the best available course of therapy with regard to content (musical components), mode of engagement (active or passive protocols), and duration and intensity (dosage) of the intervention ( Wheeler, 2015 ).
Dosage is a fundamentally important aspect to consider when studying MBIs. Regarding the question of dosage, consider the analogous case of physical activity: the American Academy of Pediatrics recommends 60 min of activity per day in school-aged children ( www.h ealthychildren.org ), and the Global Council of Brain Health recommends to “strive for at least 150 min of weekly, moderate-intensity aerobic activity” for adults over age 50 to manage heart and brain health. Is there an equivalent “recommended dosage” for music-based interventions? The answer to this question is complex, as the experience of music itself is complex. Although every society has music, the musical cultures that societies around the world have evolved are diverse and variable ( Savage et al., 2015 ). Even the same piece of music may elicit varying responses among individuals within the same culture, or for the same individual with repeated listening over time ( Margulis, 2014 ). As such, music that has therapeutic benefits for one individual may not necessarily translate to another.
In this regard, neuroscience can inform the question of dosage in MBIs by quantifying the effects of receptive music (perception) and active music (production) interventions on the CNS. For instance, fMRI studies have shown that listening to self-selected music engages the auditory and reward systems more than music selected by the researcher ( Pereira et al., 2011 ; Quinci et al., 2022 ), converging with the intuitions from music therapy. Longitudinal fMRI results in healthy older adults show that an 8 week receptive MBI increased functional connectivity from the auditory cortex to the reward system, specifically to the mPFC ( Quinci et al., 2022 ). While these results remain to be further validated with control interventions that isolate the active ingredient of music listening, the idea that systematic engagement with music can change the connectivity of the auditory and reward systems is appealing because it offers a tractable method by which to quantify the impact of MBI dosage. While specific parameters of the dose–response relationship between music and health are too complex to be knowable at this time, the responsivity, sensitivity, and connectivity of the engaged brain circuits may serve as potentially viable quantitative measures for the dose–response relationship, thus offering a window of opportunity to dissect the complexity of MBIs and their impacts on brain and health in general.
Comparative studies of musical rhythm perception
Among musical constituents, rhythm and temporal periodicity (sonic patterns which repeat regularly in time) are widely seen across species and have been richly studied. In humans, musical rhythm perception involves detecting such periodicities and generating precise temporal predictions about upcoming events ( Merchant et al., 2015 ). This ability to detect and predict auditory rhythms is central to music's positive effect on a variety of neurologic disorders involving motor functions, including normalizing gait in PD ( Benoit et al., 2014 ; Ghai et al., 2018 ; Krotinger and Loui, 2021 ), enhancing language recovery after stroke ( Schlaug et al., 2009 ; Zumbansen et al., 2014 ), and improving phonological processing in dyslexia ( Flaugnacco et al., 2015 ). While much remains to be understood about the neural mechanisms of rhythm perception, progress on this front has been facilitated by cross-species studies of perception along with incorporation of quantitative assessment and manipulation of neural activity.
Recent work has begun to elucidate the neural circuits for recognizing rhythmic communication signals based on tempo. For example, female field crickets are attracted to male calling songs within a narrow range of pulse rates, and this selectivity is mediated by a network of interneurons that processes instantaneous pulse rate using a coincidence detection mechanism ( Schoneich, 2020 ). While preference for pulse rate is hard-wired in many invertebrates, experience can shape neural responses to call rates in other species. For example, excitatory neurons in a mouse auditory cortex are innately sensitive to the most common rate of pup distress calls (∼5 syll/s), but their tuning can broaden to a wider range of rates following cohousing with pups producing a range of call rates ( Schiavo et al., 2020 ).
Moving beyond tempo, several studies have shown that auditory responses can be modulated by the presence of rhythmic patterns. For example, in gerbils, responses of neurons in the inferior colliculus are greater for noise bursts that occur on the beat of complex rhythms compared with the same bursts off the beat ( Rajendran et al., 2017 ). In mice, excitatory neurons in the auditory cortex integrate signals over longer timescales and distinguish between rhythmically structured and irregular sequences by adjusting spike timing ( Asokan et al., 2021 ). Moreover, in monkeys, EEG recordings have shown that deviant sounds elicit a larger auditory mismatch negativity signal when they are embedded in isochronous versus randomly timed sequences ( Honing et al., 2018 ).
While such studies demonstrate context-dependent modulation of neural activity in auditory regions, there is growing evidence that human rhythm perception relies on interactions between auditory and motor regions, even in the absence of movement. As discussed later in this review, neuroimaging studies have shown that activity in several motor planning regions, including the premotor cortex, supplementary motor area, and basal ganglia, is greater when a stimulus has a strong periodic pulse, or beat ( Grahn and Brett, 2007 ; Kung et al., 2013 ; Kasdan et al., 2022 ). In addition, transient disruption of auditory-motor connections using TMS can disrupt beat perception in humans without affecting perception of the timing of absolute intervals ( Ross et al., 2018 ). Together, these results support the hypothesis that perception of temporal regularity depends on the interaction of auditory and motor regions.
Investigation of the functional contribution of motor regions to auditory rhythm perception would benefit greatly from a small animal model that (1) possesses reciprocally connected auditory-motor circuitry; and (2) can recognize rhythmic patterns. Like humans, songbirds possess specialized auditory-motor circuits for learning and producing rhythmically patterned sequences ( Norton and Scharff, 2016 ; Roeske et al., 2020 ). Anatomical, physiological, and histochemical studies have found remarkable similarities in the premotor, auditory, and basal ganglia circuitry of birds and mammals, including shared cell types, patterns of connectivity, electrophysiological properties, and laminar organization ( Doupe et al., 2005 ; Goldberg and Fee, 2010 ; Goldberg et al., 2010 ; Wang et al., 2010 ). A recent study found that zebra finches, the most commonly studied songbird, can detect temporal regularities in auditory sequences and predict the timing of calls of a vocal partner, allowing them to adjust the timing of their own answers to avoid overlap ( Benichov et al., 2016 ). This ability to predictively adjust call timing was disrupted by lesions of vocal motor regions, consistent with the idea that call timing plasticity depends on the interaction of forebrain motor and auditory regions. However, it remains unclear whether zebra finches can perceive rhythms holistically or whether they learned the specific time interval between the vocal partner's calls and their own.
To examine whether songbirds can perceive rhythms holistically, as humans do, a behavioral paradigm to test whether zebra finches can learn to recognize a fundamental rhythmic pattern, equal timing between events, or “isochrony,” has been developed ( Rouse et al., 2021 ). Humans readily recognize isochrony across a wide range of rates ( Espinoza-Monroy and de Lafuente, 2021 ), indicating a facility with perceiving the relative timing of events, not just absolute interval durations. Using a sequential training procedure, whether zebra finches could discriminate between isochronous and arrhythmic sequences of a repeated song element was probed. By varying sound element identity and tempo across stimuli, birds were incentivized to attend to the relative timing in auditory sequences, rather than to specific spectral features or interval durations ( Rouse et al., 2021 ). Once birds reached a performance criterion for overall accuracy, they were tested for the ability to generalize the discrimination to stimuli at novel tempi. This study found that zebra finches, like humans, can robustly recognize isochrony across a broad range of rates, including rates 20% slower and 25% faster than the original training stimuli. Notably, birds that successfully discriminated isochronous from arrhythmic stimuli listened to more intervals before responding than birds that failed, suggesting that success at rhythm discrimination is related to attention to global temporal patterns. This aligns with evidence from neuropsychology studies showing that neural mechanisms underlying detection of relative timing are distinct from those involved in encoding absolute timing ( Grube et al., 2010 ; Teki et al., 2011 ; Breska and Ivry, 2018 ).
The finding that zebra finches, like humans, can categorize rhythms based on global temporal patterns contrasts with prior work in vocal nonlearners. For example, rats can be trained to discriminate isochronous from arrhythmic rhythms but show weak generalization when tested with stimuli at novel tempi, suggesting a strong reliance on absolute timing for rhythm perception ( Celma-Miralles and Toro, 2020 ). Thus, the combination of a well-defined auditory-motor circuit and the ability to recognize relative timing make songbirds a tractable small animal model to investigate the contributions of motor regions to detecting temporal periodicity and predicting the timing of upcoming events, two hallmarks of rhythm perception in humans. Future experiments manipulating neural activity can test for a causal role of forebrain motor regions in the perception of rhythmic patterns independent of rate, and neural recordings will help to reveal whether predictive activity emerges in motor regions as birds learn to discriminate isochronous from arrhythmic stimuli. More generally, such mechanistic studies of auditory-motor interactions during rhythm perception should help to inform music-based interventions for enhancing function in normal and disease states.
Music and motor circuits
Similar to the animal species discussed earlier, humans have a special way of perceptually and motorically interacting with rhythmic stimuli. Repeating patterns of beats, or meter, establish a temporal scaffolding that shapes future expectations, shapes the perceptual meaning of individual events, and enables behavioral synchronization among groups of people.
One of the more intriguing ideas emerging from the field is that the motor system may be important for the ordered perception of musical structure, even in the absence of overt movement ( Repp, 2005 ; Schubotz, 2007 ; Zatorre et al., 2007 ; Arnal, 2012 ; Patel and Iversen, 2014 ; Ross et al., 2016 ; Rimmele et al., 2018 ). Many accounts, such as the Action Simulation for Auditory Perception (ASAP), emphasize the role of the motor system as a source for generating temporal expectations about upcoming events, a critical biological function, specifically hypothesizing motor to auditory connectivity ( Patel and Iversen, 2014 ; Cannon and Patel, 2021 ). Recent work has specifically examined the motor system's involvement in shaping the perception and imagery of auditory rhythm in the absence of movement, directly testing the predictions of the ASAP and other motor hypotheses and providing insight into temporal perception using advanced EEG and Mobile Brain/Body Imaging (MoBI) methods. MoBI is a new imaging approach using mobile brain imaging methods, including the EEG and/or near infrared spectroscopy synchronized to body motion capture and other behavioral and psychophysiological data streams to investigate brain activity supporting participants actively interacting with their environment and/or with others ( Makeig et al., 2009 ; Gramann et al., 2011 , 2014 ).
EEG measures voltages present at the scalp as a result of dynamic electrical activity in the brain, but any single electrode measures the sum of activity from many regions of the cortex. One solution is independent component analysis ( Makeig et al., 1996 ), a method to optimally unmix the scalp signals and identify putative cortical sources. A recent study used auditory and motor localizer trials and independent component analysis to identify the most unimodal auditory and motor independent components in human participants and then examined auditory-motor interactions during a meter imagery task ( Cheng et al., 2022 ). Participants first heard an unaccented control series of drum strokes, followed by accented strokes that established a duple (1-2) or triple (1-2-3) meter, followed again by unaccented strokes with the instruction to continue imagining the previously established meter. To verify the imagery task, participants finally tapped the meter they had imagined. By comparing brain activity during the unaccented control and the meter imagery conditions, two predictions of ASAP were confirmed: (1) representation of meter was present in brain signals during meter imagery in both auditory and motor regions; and (2) robust, bidirectional motor to auditory connectivity (assessed using a directional measure of “causal” influence) ( Korzeniewska et al., 2008 ) was present during imagery. A problematic potential confound for any work on imagery is the presence of possibly unintentional, subtle movements, which can be ruled out by using MoBI methods, including motion capture and the measurement of muscle potentials. The use of neurostimulation to causally manipulate brain activity makes it possible to directly test the importance of the motor system for auditory perception. ASAP proposes a specific pathway to mediate auditory/motor reciprocal interactions, the dorsal auditory stream linking auditory cortex to premotor cortex via parietal cortex ( Rauschecker, 2011 ). One can predict that interruption of this pathway would disrupt beat perception. TMS is one method by which activity on localized cortical regions can be temporarily suppressed (e.g., using continuous theta-burst stimulation) ( Huang et al., 2005 ). Continuous theta-burst stimulation over parietal cortex has been shown to impact aspects of beat perception but spare other forms of temporal processing, providing causal evidence in favor of ASAP ( Ross et al., 2018 ).
While it is common to think of music as an auditory phenomenon, something we can thoroughly enjoy through headphones, music and movement are inseparable in several ways. Until the advent of recorded music, all music was created by movement. Many types of music strongly compel movement and dance, an aspect that has been used profitably in therapies for movement disorders. One example may be active MBIs that take advantage of musical groove, which is the pleasurable urge to move to music, possibly through connections to broader neural circuits, including the motor systems. The experience of groove is strongest for slight violations to rhythmic structure ( Janata et al., 2012 ; Witek et al., 2014 ), and is causally linked to sensorimotor coupling as demonstrated by TMS studies ( Stupacher et al., 2013 ). As such, the use of groovy music to motivate dance may be an important ingredient in active MBIs for movement disorders, such as PD, which is associated with a loss of internal cues for timing and movement, as evidenced by impaired rhythm discrimination in PD patients ( Grahn and Brett, 2009 ). As music and dance engage sensorimotor coupling through rhythm and groove, this has inspired interventions, such as Dance for PD, which is a program that uses dance as an intervention for individuals with PD and their caregivers. Standardized neurologic pre-post testing showed a reduction of Parkinsonian symptoms following 4 months of Dance for PD, with better improvement observed for those who were more accurate at finger-tapping in rhythm to music, suggesting more accurate sensorimotor coupling ( Krotinger and Loui, 2021 ). The presence of lifelong dance experience was also associated with greater reductions of both motor and nonmotor symptoms after dance intervention, suggesting that long-term training engages the predictive processes that may underlie more efficient sensorimotor coupling, which may have synergistic benefits for active MBIs.
In the past 15 years, the field of MoBI has emphasized the study of brain activity underlying active and naturalistic interactions with the environment and with others, using mobile neuroimaging methods, such as EEG, to measure brain dynamics synchronized to full-body motion capture and other behavioral and psychophysiological data streams ( Makeig et al., 2009 ). The approach is particularly attractive in therapeutic and at-home contexts because of the emerging availability of low-cost and portable EEG systems, simple camera-based motion capture, and low-cost wrist-worn physiological sensors. The study of music is a natural fit to MoBI, which has been successfully applied to studies of individual and group musical behavior ( Maidhof et al., 2014 ) toward understanding of interpersonal interactions among performers ( Chang et al., 2018 ; Varlet et al., 2020 ), between performer and audience ( Swarbrick et al., 2018 ), and among audience listeners and dancers. Cooperative musical and dance interactions involve developing trust ( Stupacher et al., 2013 ; Trainor and Cirelli, 2015 ), are positively related to interpersonal empathy ( Novembre et al., 2019 ), and can be effective for the communication of intentions and emotion through movement ( Leslie et al., 2014 ). A recent study showed that cooperative musical interactions led to long-lasting changes in interbrain phase coherence ( Khalil et al., 2022 ), perhaps indicative of a lasting cooperative set. These relationships underlie aesthetic interactions but also therapeutic ones; thus, this line of work may have broader implications for the understanding of how to create and evaluate effective therapeutic interactions in general ( Foubert et al., 2021 ). By enabling low-cost and portable assessment of brain and body states, MoBI methods will improve understanding of the mechanisms by which ecologically complex MBIs achieve therapeutic goals. These methods will also open the way for exciting new modalities of real time brain/body feedback for rehabilitative and augmentative training ( Blanco and Ramirez, 2019 ; Turner et al., 2021 ).
Music and reward circuits
The reward system consists of various neural structures, including the midbrain tegmentum, the striatum in the basal forebrain, the ventromedial and orbitofrontal cortex, and various other regions all interconnected in complex ways ( Haber, 2017 ). It plays a role in many basic biological functions and is thought to underlie our experience of hedonic pleasure ( Berridge and Kringelbach, 2015 ). Yet, until about two decades ago, it was not even known whether the pleasure generated by music was mediated by this same system; indeed, some philosophical traditions argued strongly against it ( Skov and Nadal, 2020 ).
A series of neuroimaging studies has shown that the striatum and related structures become activated when people experience pleasure from music, and that these responses scale with the degree of musical pleasure experienced ( Blood and Zatorre, 2001 ; Koelsch et al., 2006 ; Montag et al., 2011 ; Salimpoor et al., 2013 ; Matthews et al., 2020 ), as summarized in a recent a meta-analysis ( Mas-Herrero et al., 2021b ). Furthermore, psychophysiological indices of autonomic system engagement (heart rate, skin conductance, respiration, etc.) also increase with subjective reports of musical pleasure ( Grewe et al., 2007 ; Salimpoor et al., 2009 ). In addition, the laboratory of R.J.Z. showed that the striatal response is dopaminergic in nature ( Salimpoor et al., 2011 ; Ferreri et al., 2019 ), and that it is related to reward prediction mechanisms ( Gold et al., 2019 ), thus linking musical engagement of the reward system to the extensive animal literature on dopaminergic mediation of reward prediction error ( Schultz, 2016 ).
These findings were critical in setting the stage for a scientific understanding of music's effects but do not on their own provide a functional model of how music activates the reward system. Furthermore, imaging studies are necessarily correlational in nature, so causal evidence was required to really prove the point. Recent advances have addressed both these issues.
Several studies have shown that, as the subjective liking of music increases, the functional connectivity between auditory cortical systems and reward structures also increases ( Salimpoor et al., 2013 ; Shany et al., 2019 ; Quinci et al., 2022 ). This idea is very important as it suggests that patterns of sound processed in the auditory system are assigned value within the reward system. More specifically, sensory prediction errors computed in auditory cortical networks are believed to be propagated to the reward system where they are assigned value according to a reward prediction mechanism ( Zatorre, 2023 ). Thus, according to this model, musical pleasure would arise from the crosstalk between these two systems.
If this idea is correct, it leads to the prediction that people with little or no hedonic response to music should exhibit reduced interactions between the two systems. This is precisely what was observed in a series of experiments exploring specific musical anhedonia, defined as a condition in which individuals experience very little pleasure to music, yet have no perceptual deficit, nor any generalized depression or anhedonia ( Mas-Herrero et al., 2014 ). When tested with functional imaging, people with musical anhedonia showed reduced functional connectivity between auditory and striatal areas compared with average listeners and also compared with “hyperhedonic” music lovers, who showed the greatest degree of functional interaction ( Martinez-Molina et al., 2016 ). Furthermore, structural imaging of musically anhedonic people showed evidence of reduced anatomic connectivity in auditory-orbitofrontal white-matter tracts ( Martinez-Molina et al., 2019 ).
These findings strongly support the auditory-reward interaction model of musical pleasure. But more direct causal evidence was still lacking. If musical pleasure arises from these interactions, modulations of this auditory-reward circuit ought to lead to modifications of the experience of pleasure. To do so requires a method that allows for stimulation of deep structures. TMS is typically used to modulate cortical structures but can also be used to influence the striatum via targeting of dorsolateral frontal areas that are connected to it ( Strafella et al., 2001 ). Importantly, depending on the parameters of stimulation, it is possible both to upregulate dopamine activity in the striatum or downregulate it ( Pogarell et al., 2007 ; Ko et al., 2008 ).
A recent study combined these brain stimulation methods with the music-induced pleasure measures (behavioral and psychophysiological) already validated in the neuroimaging studies described above ( Mas-Herrero et al., 2018 ). The results clearly showed that, after receiving excitatory TMS (compared with sham) targeting dorsolateral frontal cortex, listeners reported higher subjective rankings of music-induced pleasure, as well as higher objective psychophysiological responses; conversely, after inhibitory TMS, both types of dependent variables were reduced. The finding that the degree of pleasure we feel can be modulated in either direction by transiently changing the excitability of certain brain structures fits the predictions of the model very well. But the final step in the logical chain would require that a direct link be shown between modulation of auditory-reward connectivity and modulation of music-induced pleasure.
To achieve this goal, Mas-Herrero et al. (2021a ) repeated the TMS experiment with a new sample of volunteers and different musical excerpts, but this time fMRI was acquired immediately after the TMS session, to document the neural changes associated with the stimulation. The behavioral findings from this study mirrored those from the previous one, showing that the stimulation effects are robust and replicable. The most important finding from the fMRI data were that the functional connectivity between the right auditory cortex and the right ventral striatum was modulated in direct relationship with the degree to which the stimulation changed pleasure ratings. Thus, enhancement or decrement in pleasure following stimulation was related to upregulation or downregulation of the auditory-reward circuitry. This outcome thus provides definitive, causal evidence in favor of the hypothesis that these interactions underlie the experience of musical pleasure.
Although much remains to be discovered, these experimental findings, together, provide a mechanistic understanding of the neural basis of music-induced pleasure. Such basic-science knowledge is essential to move forward with potential applications of music to various disorders. Indeed, we know that affective states can be manipulated via music (including mood induction or emotion regulation, for example). It is likely that the reward system plays a key role in these functions, which is consistent with the view of music as a transformative technology of the mind ( Patel, 2008 , 2018 ; Loughridge, 2021 ) in the sense that music both emerges as a creative product of the mind and can shape the mind by affecting its function. This underlying mechanism may therefore explain why music can be used to improve mood, reduce anxiety, and enhance well-being in many different clinical groups, including psychiatric disorders ( Gebhardt et al., 2014 ), depression ( Maratos et al., 2011 ), stroke ( Särkämö et al., 2008 ), heart disease ( Bradt et al., 2013 ), and dementia ( Guétin et al., 2011 ; for systematic reviews and meta-analysis, see Sihvonen et al., 2017 ; de Witte et al., 2020 ).
Music and cognitive and sensory circuits
The relationships between music and cognitive or sensory functions have been explored largely in human subjects. Longitudinal studies have shown that multiple years of music education or training are associated with enhanced executive functions, including inhibition, planning, and verbal intelligence in school-aged children ( Jaschke et al., 2018 ; Hennessy et al., 2019 ). Similarly in older adults, musical practice has also been shown to benefit cognitive function ( Roman-Caballero et al., 2018 ); while various forms of MBIs seem to benefit cognitive functions, including short-term and working memories, digit span, orientation, fluency, abstraction, and psychomotor speed, as well as reduce pain in people with dementia ( Hofbauer et al., 2022 ). A substantial amount of literature can also be found to support music as an adjuvant pain treatment ( Lunde et al., 2019 ). In contrast, relatively few studies focus on the brain mechanisms by which MBIs deliver their cognitive and sensory effects in humans ( Chaddock-Heyman et al., 2021 ) or in an animal model ( Zhou et al., 2022 ).
One concept for music to shape cognitive circuits lies in its ability to engender predictions ( Vuust et al., 2022 ): as we become exposed to musical sounds throughout the lifespan, the brain continuously and automatically learns to form predictions for sounds that will likely come next, and the implicit learning of these predictions and minimization of prediction errors shapes the cognitive circuits that give rise to one's body of knowledge, including of music within the culture. As a concrete example of these cognitive circuits at work, most listeners within the Western culture show implicit knowledge of musical scale: in common-practice Western music, musical scales are based around the octave, which is a doubling of acoustic frequency. This knowledge is based on exposure to the environment through one's culture, and the ability to learn from the environment via statistical learning as a cognitive mechanism is key among the cognitive circuits that give rise to musical knowledge. To test the cognitive mechanism of statistical learning outside of Western culture, Loui and colleagues ( Loui et al., 2010 ; Loui, 2022 ) used digital musical technology to create music in the Bohlen–Pierce scale, which is an alternative tuning system based on a tripling of acoustic frequency. Systematically manipulating predictions for music composed in this new scale affected liking of the new music: more frequently presented patterns were more preferred, suggesting a dose–response type relationship between familiarity and preference. The statistical learning of predictions was comparable across U.S. and Chinese populations, suggesting a relatively similar dose–response relationship across cultures. Furthermore, functional neuroimaging showed that statistical learning of predictions was tied to the activity and connectivity of the auditory and reward systems ( Kathios et al., 2022 ). Together, these results underlie the idea that prediction and reward may be a cognitive mechanism that explains how musical sounds become rewarding. Future work is needed to relate prediction and reward learning to the transfer effects of musical experiences toward more domain-general cognitive functions, which may in turn underlie the success of MBIs for multiple clinical populations.
Collectively, this review aims to highlight a few approaches and examples of studies on music and a variety of brain circuits, rather than attempt to be comprehensive in the entire literature covering music and neuroscience. As we study music in the context of brain and health, thus studying music as an intervention, it is important to clearly define and describe the basic contents of an MBI, either including the chosen melody, harmony, and rhythm at the minimum, or articulating the contents of the self-selected music at the onset of a study. Whether an MBI is delivered in a receptive mode to a study subject by passively listening to the musical content or in an engaging active mode with the study subject participating in producing the musical content, or both, should also be clearly specified, in addition to whether an MBI involves a group setting, either as a group listening or performing event or as an interaction between therapists, teachers, or performers and patients, students, or audience. Clear specifications of the musical content and delivery mode can enable a better design of the control interventions as often required in rigorously designed mechanistic and clinical studies. Like all other types of intervention studies, determining the dosages, including intensity of the music contents as well as the frequency and duration of the delivery, should be a fundamental requirement for MBI studies.
In terms of comparative studies of MBIs, this review has emphasized the power of animal models in vocal learning species, such as songbirds, in elucidating auditory-motor interactions in rhythm perception, which may ultimately help us understand how and why periodic auditory rhythms can help normalize motor function in disorders, such as stroke and PD. Animal models are also critical for understanding interactions between auditory regions and other neural circuits, including the reward system and pathways for detecting and coding noxious stimuli that cause pain. For example, work in songbirds has begun to elucidate auditory-reward interactions. In male birds, dopaminergic neurons are sensitive to the quality of the bird's own vocal performance, exhibiting differential firing rates when song performance is better or worse than expected (“reward prediction error”) ( Gadagkar et al., 2016 ). Similarly, recent work in mice has begun to shed light on the neural mechanisms underlying the ability of music to attenuate pain intensity in humans ( Garza-Villarreal et al., 2017 ). Using a mouse model for peripheral pain, Zhou et al. (2022) found that sound (and potentially music) presented at a level slightly above background noise can blunt behavioral signs of pain by modulating cortico-thalamic input from auditory regions to posterior and ventral posterior nuclei of the thalamus. This effect can last for several days after sound exposure, so it cannot be explained by sound's short-term effect on attention. Together, these studies highlight the utility of animal models for investigating the mechanistic underpinnings of both active and receptive MBIs. Future endeavors captalizing on the power of molecular genetics in combination with sophisticated behavioral assays to probe motor, affective, cognitive, and sensory systems, such as audition, pain, interoception, and vision, may facilitate the discovery of novel mechanistic insights into MBIs.
In human brain circuit studies, EEG, MEG, and fMRI have helped to elucidate neural networks involved in MBIs. Much of the brain imaging evidence has supported the engagement of auditory, motor, and reward/affective neural circuits, some of which is further enhanced evidence provided by brain stimulation studies using technologies, such as TMS and high-resolution behavioral data collected by cutting-edge motion capture technologies. The evidence for engagement of cognitive and other sensory brain circuits unique to MBIs has, however, been relatively scant. Future studies incorporating powerful brain imaging and stimulation technologies and novel behavioral assessments may be needed to ascertain whether cognitive and other sensory circuits are also engaged during MBIs in human populations.
In conclusion, the complexity of MBIs and their potential impact on multiple brain circuits may require sophisticated computational approaches to further mechanistic understandings. Predication analysis, already applied for studying the relationships between music and motor, reward, and cognitive circuits, is a well-tested example of how a computational approach may enhance mechanistic insights. Development of cutting-edge computational tools, including machine learning methods, may help inform evidence-based research of MBIs in the future.
We thank Karen Kaplan and Catherine Law for help in overall edits to improve this manuscript; and Bryan Ewsichek for creating Figure 1 .
The authors declare no competing financial interests.
- Arnal LH (2012) Predicting 'when' using the motor system's beta-band oscillations . Front Hum Neurosci 6 :225. 10.3389/fnhum.2012.00225 [ PMC free article ] [ PubMed ] [ CrossRef ] [ Google Scholar ]
- Asokan MM, Williamson RS, Hancock KE, Polley DB (2021) Inverted central auditory hierarchies for encoding local intervals and global temporal patterns . Curr Biol 31 :1762–1770.e4. 10.1016/j.cub.2021.01.076 [ PMC free article ] [ PubMed ] [ CrossRef ] [ Google Scholar ]
- Benichov JI, Benezra SE, Vallentin D, Globerson E, Long MA, Tchernichovski O (2016) The forebrain song system mediates predictive call timing in female and male zebra finches . Curr Biol 26 :309–318. 10.1016/j.cub.2015.12.037 [ PMC free article ] [ PubMed ] [ CrossRef ] [ Google Scholar ]
- Benoit CE, Dalla Bella S, Farrugia N, Obrig H, Mainka S, Kotz SA (2014) Musically cued gait-training improves both perceptual and motor timing in Parkinson's disease . Front Hum Neurosci 8 :494. [ PMC free article ] [ PubMed ] [ Google Scholar ]
- Berridge KC, Kringelbach ML (2015) Pleasure systems in the brain . Neuron 86 :646–664. 10.1016/j.neuron.2015.02.018 [ PMC free article ] [ PubMed ] [ CrossRef ] [ Google Scholar ]
- Blanco AD, Ramirez R (2019) Evaluation of a sound quality visual feedback system for bow learning technique in violin beginners: an EEG study . Front Psychol 10 :165. 10.3389/fpsyg.2019.00165 [ PMC free article ] [ PubMed ] [ CrossRef ] [ Google Scholar ]
- Blood AJ, Zatorre RJ (2001) Intensely pleasurable responses to music correlate with activity in brain regions implicated in reward and emotion . Proc Natl Acad Sci USA 98 :11818–11823. 10.1073/pnas.191355898 [ PMC free article ] [ PubMed ] [ CrossRef ] [ Google Scholar ]
- Bradt J, Dileo C, Potvin N (2013) Music for stress and anxiety reduction in coronary heart disease patients . Cochrane Database Syst Rev 12 : CD006577. [ PMC free article ] [ PubMed ] [ Google Scholar ]
- Breska A, Ivry RB (2018) Double dissociation of single-interval and rhythmic temporal prediction in cerebellar degeneration and Parkinson's disease . Proc Natl Acad Sci USA 115 :12283–12288. 10.1073/pnas.1810596115 [ PMC free article ] [ PubMed ] [ CrossRef ] [ Google Scholar ]
- Cannon JJ, Patel AD (2021) How beat perception co-opts motor neurophysiology . Trends Cogn Sci 25 :137–150. 10.1016/j.tics.2020.11.002 [ PMC free article ] [ PubMed ] [ CrossRef ] [ Google Scholar ]
- Celma-Miralles A, Toro JM (2020) Discrimination of temporal regularity in rats ( Rattus norvegicus ) and humans ( Homo sapiens ) . J Comp Psychol 134 :3–10. 10.1037/com0000202 [ PubMed ] [ CrossRef ] [ Google Scholar ]
- Chaddock-Heyman L, Loui P, Weng TB, Weisshappel R, McAuley E, Kramer AF (2021) Musical training and brain volume in older adults . Brain Sci 11 :50. [ PMC free article ] [ PubMed ] [ Google Scholar ]
- Chang A, Bosnyak DJ, Trainor LJ (2018) Beta oscillatory power modulation reflects the predictability of pitch change . Cortex 106 :248–260. 10.1016/j.cortex.2018.06.008 [ PubMed ] [ CrossRef ] [ Google Scholar ]
- Cheever T, Taylor A, Finkelstein R, Edwards E, Thomas L, Bradt J, Holochwost SJ, Johnson JK, Limb C, Patel AD, Tottenham N, Iyengar S, Rutter D, Fleming R, Collins FS (2018) NIH/Kennedy Center Workshop on Music and the Brain: Finding Harmony . Neuron 97 :1214–1218. 10.1016/j.neuron.2018.02.004 [ PMC free article ] [ PubMed ] [ CrossRef ] [ Google Scholar ]
- Chen WG, Riddle R, Onken L, Cui C, Poremba A, Hillefors M, Scholoesser DM (2018) Promoting research on music and health: phased innovation award for music interventions (R61/R33, Clinical Trial Optional) . NIH Funding Opportunity Announcement RFA-AT-19-001 . [ Google Scholar ]
- Chen WG, St. Hillaire-Clarke C, Onken L, Bakos A, Xu B, Leitman DI (2020) Promoting research on music and health: phased innovation award for music interventions (R61/R33 Clinical Trial Optional) . NIH Funding Opportunity Announcement PAR-20-266 . [ Google Scholar ]
- Chen WG, Schloesser D, Arensdorf AM, Simmons JM, Cui C, Valentino R, Gnadt JW, Nielsen L, Hillaire-Clarke CS, Spruance V, Horowitz TS, Vallejo YF, Langevin HM (2021) The emerging science of interoception: sensing, integrating, interpreting, and regulating signals within the self . Trends Neurosci 44 :3–16. 10.1016/j.tins.2020.10.007 [ PMC free article ] [ PubMed ] [ CrossRef ] [ Google Scholar ]
- Cheng TH, Creel SC, Iversen JR (2022) How do you feel the rhythm? Dynamic motor-auditory interactions are involved in the imagination of hierarchical timing . J Neurosci 42 :500–512. 10.1523/JNEUROSCI.1121-21.2021 [ PMC free article ] [ PubMed ] [ CrossRef ] [ Google Scholar ]
- de Witte M, Spruit A, van Hooren S, Moonen X, Stams GJ (2020) Effects of music interventions on stress-related outcomes: a systematic review and two meta-analyses . Health Psychol Rev 14 :294–324. 10.1080/17437199.2019.1627897 [ PubMed ] [ CrossRef ] [ Google Scholar ]
- Doupe AJ, Perkel DJ, Reiner A, Stern EA (2005) Birdbrains could teach basal ganglia research a new song . Trends Neurosci 28 :353–363. 10.1016/j.tins.2005.05.005 [ PubMed ] [ CrossRef ] [ Google Scholar ]
- Edwards E, St. Hilliaire-Clarke C., Frank DW, Finkelstein R, Cheever T, Chen WG, Onken L, Poremba A, Riddle R, Schloesser DM, Burgdort C, Wells N, Fleming R, Collins FS (2022) The NIH Music-Based Interventions Toolkit . Neurology , in press. [ Google Scholar ]
- Espinoza-Monroy M, de Lafuente V (2021) Discrimination of regular and irregular rhythms explained by a time difference accumulation model . Neuroscience 459 :16–26. 10.1016/j.neuroscience.2021.01.035 [ PubMed ] [ CrossRef ] [ Google Scholar ]
- Ferreri L, Mas-Herrero E, Zatorre RJ, Ripolles P, Gomez-Andres A, Alicart H, Olive G, Marco-Pallares J, Antonijoan RM, Valle M, Riba J, Rodriguez-Fornells A (2019) Dopamine modulates the reward experiences elicited by music . Proc Natl Acad Sci USA 116 :3793–3798. 10.1073/pnas.1811878116 [ PMC free article ] [ PubMed ] [ CrossRef ] [ Google Scholar ]
- Flaugnacco E, Lopez L, Terribili C, Montico M, Zoia S, Schon D (2015) Music training increases phonological awareness and reading skills in developmental dyslexia: a randomized control trial . PLoS One 10 :e0138715. 10.1371/journal.pone.0138715 [ PMC free article ] [ PubMed ] [ CrossRef ] [ Google Scholar ]
- Foubert K, Gill SP, De Backer J (2021) A musical improvisation framework for Shaping Interpersonal Trust . Nordic J Music Ther 30 :79–96. 10.1080/08098131.2020.1788627 [ CrossRef ] [ Google Scholar ]
- Gadagkar V, Puzerey PA, Chen R, Baird-Daniel E, Farhang AR, Goldberg JH (2016) Dopamine neurons encode performance error in singing birds . Science 354 :1278–1282. 10.1126/science.aah6837 [ PMC free article ] [ PubMed ] [ CrossRef ] [ Google Scholar ]
- Garza-Villarreal EA, Chakravarty MM, Hansen B, Eskildsen SF, Devenyi GA, Castillo-Padilla D, Balducci T, Reyes-Zamorano E, Jespersen SN, Perez-Palacios P, Patel R, Gonzalez-Olvera JJ (2017) The effect of crack cocaine addiction and age on the microstructure and morphology of the human striatum and thalamus using shape analysis and fast diffusion kurtosis imaging . Transl Psychiatry 7 :e1122. 10.1038/tp.2017.92 [ PMC free article ] [ PubMed ] [ CrossRef ] [ Google Scholar ]
- Gebhardt S, Kunkel M, Georgi R (2014) Emotion modulation in psychiatric patients through music . Music Percept 31 :485–493. 10.1525/mp.2014.31.5.485 [ CrossRef ] [ Google Scholar ]
- Ghai S, Ghai I, Schmitz G, Effenberg AO (2018) Effect of rhythmic auditory cueing on parkinsonian gait: a systematic review and meta-analysis . Sci Rep 8 :506. 10.1038/s41598-017-16232-5 [ PMC free article ] [ PubMed ] [ CrossRef ] [ Google Scholar ]
- Gold BP, Mas-Herrero E, Zeighami Y, Benovoy M, Dagher A, Zatorre RJ (2019) Musical reward prediction errors engage the nucleus accumbens and motivate learning . Proc Natl Acad Sci USA 116 :3310–3315. 10.1073/pnas.1809855116 [ PMC free article ] [ PubMed ] [ CrossRef ] [ Google Scholar ]
- Goldberg JH, Fee MS (2010) Singing-related neural activity distinguishes four classes of putative striatal neurons in the songbird basal ganglia . J Neurophysiol 103 :2002–2014. 10.1152/jn.01038.2009 [ PMC free article ] [ PubMed ] [ CrossRef ] [ Google Scholar ]
- Goldberg JH, Adler A, Bergman H, Fee MS (2010) Singing-related neural activity distinguishes two putative pallidal cell types in the songbird basal ganglia: comparison to the primate internal and external pallidal segments . J Neurosci 30 :7088–7098. 10.1523/JNEUROSCI.0168-10.2010 [ PMC free article ] [ PubMed ] [ CrossRef ] [ Google Scholar ]
- Grahn JA, Brett M (2007) Rhythm and beat perception in motor areas of the brain . J Cogn Neurosci 19 :893–906. 10.1162/jocn.2007.19.5.893 [ PubMed ] [ CrossRef ] [ Google Scholar ]
- Grahn JA, Brett M (2009) Impairment of beat-based rhythm discrimination in Parkinson's disease . Cortex 45 :54–61. 10.1016/j.cortex.2008.01.005 [ PubMed ] [ CrossRef ] [ Google Scholar ]
- Gramann K, Ferris DP, Gwin J, Makeig S (2014) Imaging natural cognition in action . Int J Psychophysiol 91 :22–29. 10.1016/j.ijpsycho.2013.09.003 [ PMC free article ] [ PubMed ] [ CrossRef ] [ Google Scholar ]
- Gramann K, Gwin JT, Ferris DP, Oie K, Jung TP, Lin CT, Liao LD, Makeig S (2011) Cognition in action: imaging brain/body dynamics in mobile humans . Rev Neurosci 22 :593–608. 10.1515/RNS.2011.047 [ PubMed ] [ CrossRef ] [ Google Scholar ]
- Grewe O, Nagel F, Kopiez R, Altenmüller E (2007) Listening to music as a re-creative process: physiological, psychological, and psychoacoustical correlates of chills and strong emotions . Music Percept 24 :297–314. 10.1525/mp.2007.24.3.297 [ CrossRef ] [ Google Scholar ]
- Grube M, Cooper FE, Chinnery PF, Griffiths TD (2010) Dissociation of duration-based and beat-based auditory timing in cerebellar degeneration . Proc Natl Acad Sci USA 107 :11597–11601. 10.1073/pnas.0910473107 [ PMC free article ] [ PubMed ] [ CrossRef ] [ Google Scholar ]
- Guétin S, Florence P, Gabelle A, Touchon J, Bonté F (2011) Effects of music therapy on anxiety and depression in patients with Alzheimer's disease: a randomized controlled trial . Alzheimers Dement 7 :e49. [ Google Scholar ]
- Haber SN (2017) Anatomy and connectivity of the reward circuit . In: Decision Neuroscience (Dreher JC, Tremblay L, eds), pp 3–19. San Diego: Academic. [ Google Scholar ]
- Hanser SB (2016) Integrative health through music therapy: accompanying the journey from illness to wellness . New York: Springer. [ Google Scholar ]
- Hennessy SL, Sachs ME, Ilari B, Habibi A (2019) Effects of music training on inhibitory control and associated neural networks in school-aged children: a longitudinal study . Front Neurosci 13 :1080. 10.3389/fnins.2019.01080 [ PMC free article ] [ PubMed ] [ CrossRef ] [ Google Scholar ]
- Hofbauer LM, Ross SD, Rodriguez FS (2022) Music-based interventions for community-dwelling people with dementia: a systematic review . Health Soc Care Community . Advance online publication. Retrieved Jun 30, 2022. doi: 10.1111/hsc.13895. [ PubMed ] [ CrossRef ] [ Google Scholar ]
- Honing H, Bouwer FL, Prado L, Merchant H (2018) Rhesus monkeys ( Macaca mulatta ) sense isochrony in rhythm, but not the beat: additional support for the gradual audiomotor evolution hypothesis . Front Neurosci 12 :475. [ PMC free article ] [ PubMed ] [ Google Scholar ]
- Huang YZ, Edwards MJ, Rounis E, Bhatia KP, Rothwell JC (2005) Theta burst stimulation of the human motor cortex . Neuron 45 :201–206. 10.1016/j.neuron.2004.12.033 [ PubMed ] [ CrossRef ] [ Google Scholar ]
- Janata P, Tomic ST, Haberman JM (2012) Sensorimotor coupling in music and the psychology of the groove . J Exp Psychol Gen 141 :54–75. 10.1037/a0024208 [ PubMed ] [ CrossRef ] [ Google Scholar ]
- Jaschke AC, Honing H, Scherder EJA (2018) Longitudinal analysis of music education on executive functions in primary school children . Front Neurosci 12 :103. 10.3389/fnins.2018.00103 [ PMC free article ] [ PubMed ] [ CrossRef ] [ Google Scholar ]
- Kasdan AV, Burgess AN, Pizzagalli F, Scartozzi A, Chern A, Kotz SA, Wilson SM, Gordon RL (2022) Identifying a brain network for musical rhythm: a functional neuroimaging meta-analysis and systematic review . Neurosci Biobehav Rev 136 :104588. 10.1016/j.neubiorev.2022.104588 [ PMC free article ] [ PubMed ] [ CrossRef ] [ Google Scholar ]
- Kathios N, Sachs ME, Zhang E, Ou Y, Loui P (2022) Generating new musical preferences from hierarchical mapping of predictions to reward . Submitted. [ Google Scholar ]
- Khalil A, Musacchia G, Iversen JR (2022) It takes two: interpersonal neural synchrony is increased after musical interaction . Brain Sci 12 :409. 10.3390/brainsci12030409 [ PMC free article ] [ PubMed ] [ CrossRef ] [ Google Scholar ]
- Ko JH, Monchi O, Ptito A, Petrides M, Strafella AP (2008) Repetitive transcranial magnetic stimulation of dorsolateral prefrontal cortex affects performance of the Wisconsin Card Sorting Task during provision of feedback . Int J Biomed Imaging 2008 :143238. [ PMC free article ] [ PubMed ] [ Google Scholar ]
- Koelsch S, Fritz T, V Cramon DY, Müller K, Friederici AD (2006) Investigating emotion with music: an fMRI study . Hum Brain Mapp 27 :239–250. 10.1002/hbm.20180 [ PMC free article ] [ PubMed ] [ CrossRef ] [ Google Scholar ]
- Korzeniewska A, Crainiceanu CM, Kuś R, Franaszczuk PJ, Crone NE (2008) Dynamics of event-related causality in brain electrical activity . Hum Brain Mapp 29 :1170–1192. 10.1002/hbm.20458 [ PMC free article ] [ PubMed ] [ CrossRef ] [ Google Scholar ]
- Krotinger A, Loui P (2021) Rhythm and groove as cognitive mechanisms of dance intervention in Parkinson's disease . PLoS One 16 :e0249933. 10.1371/journal.pone.0249933 [ PMC free article ] [ PubMed ] [ CrossRef ] [ Google Scholar ]
- Kung SJ, Chen JL, Zatorre RJ, Penhune VB (2013) Interacting cortical and basal ganglia networks underlying finding and tapping to the musical beat . J Cogn Neurosci 25 :401–420. 10.1162/jocn_a_00325 [ PubMed ] [ CrossRef ] [ Google Scholar ]
- Leslie G, Ojeda A, Makeig SD (2014) Measuring musical engagement using expressive movement and EEG brain dynamics . Psychomusicology 24 :75–91. 10.1037/pmu0000031 [ CrossRef ] [ Google Scholar ]
- Loughridge D (2021) 'Always already technological': new views of music and the human in musicology and the cognitive sciences . Music Res Annu 2 :1–22. [ Google Scholar ]
- Loui P (2020) Neuroscientific insights for improved outcomes in music-based interventions . Music Sci 3 :205920432096506. 10.1177/2059204320965065 [ CrossRef ] [ Google Scholar ]
- Loui P (2022) New music system reveals spectral contribution to statistical learning . Cognition 224 :105071. 10.1016/j.cognition.2022.105071 [ PubMed ] [ CrossRef ] [ Google Scholar ]
- Loui P, Wessel DL, Hudson Kam CL (2010) Humans rapidly learn grammatical structure in a new musical scale . Music Percept 27 :377–388. 10.1525/mp.2010.27.5.377 [ PMC free article ] [ PubMed ] [ CrossRef ] [ Google Scholar ]
- Lunde SJ, Vuust P, Garza-Villarreal EA, Vase L (2019) Music-induced analgesia: how does music relieve pain? Pain 160 :989–993. 10.1097/j.pain.0000000000001452 [ PubMed ] [ CrossRef ] [ Google Scholar ]
- Maidhof C, Kästner T, Makkonen T (2014) Combining EEG, MIDI, and motion capture techniques for investigating musical performance . Behav Res Methods 46 :185–195. 10.3758/s13428-013-0363-9 [ PubMed ] [ CrossRef ] [ Google Scholar ]
- Makeig SD, Bell AJ, Jung TP (1996) Independent component analysis of electroencephalographic data . Adv Neural Information Process Syst 8 :145–151. [ Google Scholar ]
- Makeig SD, Gramann K, Jung TP, Sejnowski TJ, Poizner H (2009) Linking brain, mind and behavior . Int J Psychophysiol 73 :95–100. 10.1016/j.ijpsycho.2008.11.008 [ PMC free article ] [ PubMed ] [ CrossRef ] [ Google Scholar ]
- Maratos A, Crawford MJ, Procter S (2011) Music therapy for depression: it seems to work, but how? Br J Psychiatry 199 :92–93. 10.1192/bjp.bp.110.087494 [ PubMed ] [ CrossRef ] [ Google Scholar ]
- Margulis EH (2014) On repeat: how music plays the mind . Oxford: Oxford UP. [ Google Scholar ]
- Martinez-Molina N, Mas-Herrero E, Rodriguez-Fornells A, Zatorre RJ, Marco-Pallares J (2016) Neural correlates of specific musical anhedonia . Proc Natl Acad Sci USA 113 :E7337–E7345. [ PMC free article ] [ PubMed ] [ Google Scholar ]
- Martinez-Molina N, Mas-Herrero E, Rodriguez-Fornells A, Zatorre RJ, Marco-Pallares J (2019) White matter microstructure reflects individual differences in music reward sensitivity . J Neurosci 39 :5018–5027. 10.1523/JNEUROSCI.2020-18.2019 [ PMC free article ] [ PubMed ] [ CrossRef ] [ Google Scholar ]
- Mas-Herrero E, Zatorre RJ, Rodriguez-Fornells A, Marco-Pallares J (2014) Dissociation between musical and monetary reward responses in specific musical anhedonia . Curr Biol 24 :699–704. 10.1016/j.cub.2014.01.068 [ PubMed ] [ CrossRef ] [ Google Scholar ]
- Mas-Herrero E, Dagher A, Zatorre RJ (2018) Modulating musical reward sensitivity up and down with transcranial magnetic stimulation . Nat Hum Behav 2 :27–32. 10.1038/s41562-017-0241-z [ PubMed ] [ CrossRef ] [ Google Scholar ]
- Mas-Herrero E, Dagher A, Farres-Franch M, Zatorre RJ (2021a) Unraveling the temporal dynamics of reward signals in music-induced pleasure with TMS . J Neurosci 41 :3889–3899. 10.1523/JNEUROSCI.0727-20.2020 [ PMC free article ] [ PubMed ] [ CrossRef ] [ Google Scholar ]
- Mas-Herrero E, Maini L, Sescousse G, Zatorre RJ (2021b) Common and distinct neural correlates of music and food-induced pleasure: a coordinate-based meta-analysis of neuroimaging studies . Neurosci Biobehav Rev 123 :61–71. 10.1016/j.neubiorev.2020.12.008 [ PubMed ] [ CrossRef ] [ Google Scholar ]
- Matthews TE, Witek MA, Lund T, Vuust P, Penhune VB (2020) The sensation of groove engages motor and reward networks . Neuroimage 214 :116768. 10.1016/j.neuroimage.2020.116768 [ PubMed ] [ CrossRef ] [ Google Scholar ]
- Merchant H, Grahn J, Trainor L, Rohrmeier M, Fitch WT (2015) Finding the beat: a neural perspective across humans and nonhuman primates . Philos Trans R Soc Lond B Biol Sci 370 :20140093. 10.1098/rstb.2014.0093 [ PMC free article ] [ PubMed ] [ CrossRef ] [ Google Scholar ]
- Montag C, Reuter M, Axmacher N (2011) How one's favorite song activates the reward circuitry of the brain: personality matters! Behav Brain Res 225 :511–514. 10.1016/j.bbr.2011.08.012 [ PubMed ] [ CrossRef ] [ Google Scholar ]
- Norton P, Scharff C (2016) 'Bird song metronomics': isochronous organization of zebra finch song rhythm . Front Neurosci 10 :309. [ PMC free article ] [ PubMed ] [ Google Scholar ]
- Novembre G, Mitsopoulos Z, Keller PE (2019) Empathic perspective taking promotes interpersonal coordination through music . Sci Rep 9 :12255. 10.1038/s41598-019-48556-9 [ PMC free article ] [ PubMed ] [ CrossRef ] [ Google Scholar ]
- Patel AD (2008) Music as a transformative technology of the mind . In: Music: its evolution, cognitive basis, and spiritual dimensions , pp 18–20. Oxford University Press. [ Google Scholar ]
- Patel AD (2018) Music as a transformative technology of the mind: an update . The evolution of musicality (Honing H, ed) Cambridge, MA: Massachusetts Institute of Technology. [ Google Scholar ]
- Patel AD, Iversen JR (2014) The evolutionary neuroscience of musical beat perception: the Action Simulation for Auditory Prediction (ASAP) hypothesis . Front Syst Neurosci 8 :57. 10.3389/fnsys.2014.00057 [ PMC free article ] [ PubMed ] [ CrossRef ] [ Google Scholar ]
- Pereira CS, Teixeira J, Figueiredo P, Xavier J, Castro SL, Brattico E (2011) Music and emotions in the brain: familiarity matters . PLoS One 6 :e27241. 10.1371/journal.pone.0027241 [ PMC free article ] [ PubMed ] [ CrossRef ] [ Google Scholar ]
- Pogarell O, Koch W, Popperl G, Tatsch K, Jakob F, Mulert C, Grossheinrich N, Rupprecht R, Moller HJ, Hegerl U, Padberg F (2007) Acute prefrontal rTMS increases striatal dopamine to a similar degree as D-amphetamine . Psychiatry Res 156 :251–255. 10.1016/j.pscychresns.2007.05.002 [ PubMed ] [ CrossRef ] [ Google Scholar ]
- Quinci MA, Belden A, Goutama V, Gong D, Hanser S, Donovan NJ, Geddes M, Loui P (2022) Longitudinal changes in auditory and reward systems following receptive music-based intervention in older adults . Sci Rep 12 :11517. 10.1038/s41598-022-15687-5 [ PMC free article ] [ PubMed ] [ CrossRef ] [ Google Scholar ]
- Rajendran VG, Harper NS, Garcia-Lazaro JA, Lesica NA, Schnupp JW (2017) Midbrain adaptation may set the stage for the perception of musical beat . Proc Biol Sci 284 :20171455. [ PMC free article ] [ PubMed ] [ Google Scholar ]
- Rauschecker JP (2011) An expanded role for the dorsal auditory pathway in sensorimotor control and integration . Hear Res 271 :16–25. 10.1016/j.heares.2010.09.001 [ PMC free article ] [ PubMed ] [ CrossRef ] [ Google Scholar ]
- Repp BH (2005) Rate limits of on-beat and off-beat tapping with simple auditory rhythms: 2. The roles of different kinds of accent . Music Percept 23 :165–188. 10.1525/mp.2005.23.2.165 [ CrossRef ] [ Google Scholar ]
- Riddle R, Chen WG, Wiggs C, St. Hillaire-Clark C, Cui C, Poremba A, Vallejo Y, Adams L, Hillefors M, Scholoesser DM (2018a) Promoting research on music and health: fundamentals and applications (R01 Clinical Trials Optional) . NIH Funding Opportunity Announcement RFA-NS-19-008 . [ Google Scholar ]
- Riddle R, Chen WG, Wiggs C, St. Hillaire-Clark C, Cui C, Poremba A, Vallejo Y, Adams L, Hillefors M, Scholoesser DM (2018b) Promoting research on music and health: fundamentals and applications (R21 Clinical Trials Optional) . NIH Funding Opportunity Announcement RFA-NS-19-009 . [ Google Scholar ]
- Riddle R, Wiggs C, St. Hillaire-Clark C, Onken L, Xu B, Chen WG, Bakos A, Hoffman EA, Crisius M, Hillefors M, Mujuru P, Adams L, Scholoesser DM, Iyengar S (2020a) Music and health: understanding and developing music medicine (R01 Clinical Trial Optional) . NIH Funding Opportunity Announcement PAR-21-100 . [ Google Scholar ]
- Riddle R, Wiggs C, St. Hillaire-Clark C, Onken L, Xu B, Chen WG, Bakos A, Hoffman EA, Crisius M, Hillefors M, Mujuru P, Adams L, Scholoesser DM, Iyengar S (2020b) Music and health: understanding and developing music medicine (R21 Clinical Trial Optional) . NIH Funding Opportunity Announcement PAR-21-099 . [ Google Scholar ]
- Rimmele JM, Morillon B, Poeppel D, Arnal LH (2018) Proactive sensing of periodic and aperiodic auditory patterns . Trends Cogn Sci 22 :870–882. 10.1016/j.tics.2018.08.003 [ PubMed ] [ CrossRef ] [ Google Scholar ]
- Roeske TC, Tchernichovski O, Poeppel D, Jacoby N (2020) Categorical rhythms are shared between songbirds and humans . Curr Biol 30 :3544–3555.e6. 10.1016/j.cub.2020.06.072 [ PMC free article ] [ PubMed ] [ CrossRef ] [ Google Scholar ]
- Roman-Caballero R, Arnedo M, Trivino M, Lupianez J (2018) Musical practice as an enhancer of cognitive function in healthy aging: a systematic review and meta-analysis . PLoS One 13 :e0207957. 10.1371/journal.pone.0207957 [ PMC free article ] [ PubMed ] [ CrossRef ] [ Google Scholar ]
- Ross JM, Iversen JR, Balasubramaniam R (2016) Motor simulation theories of musical beat perception . Neurocase 22 :558–565. 10.1080/13554794.2016.1242756 [ PubMed ] [ CrossRef ] [ Google Scholar ]
- Ross JM, Iversen JR, Balasubramaniam R (2018) The role of posterior parietal cortex in beat-based timing perception: a continuous theta burst stimulation study . J Cogn Neurosci 30 :634–643. 10.1162/jocn_a_01237 [ PubMed ] [ CrossRef ] [ Google Scholar ]
- Rouse AA, Patel AD, Kao MH (2021) Vocal learning and flexible rhythm pattern perception are linked: evidence from songbirds . Proc Natl Acad Sci USA 118 :e2026130118. [ PMC free article ] [ PubMed ] [ Google Scholar ]
- Salimpoor VN, Benovoy M, Longo G, Cooperstock JR, Zatorre RJ (2009) The rewarding aspects of music listening are related to degree of emotional arousal . PLoS One 4 :e7487. 10.1371/journal.pone.0007487 [ PMC free article ] [ PubMed ] [ CrossRef ] [ Google Scholar ]
- Salimpoor VN, Benovoy M, Larcher K, Dagher A, Zatorre RJ (2011) Anatomically distinct dopamine release during anticipation and experience of peak emotion to music . Nat Neurosci 14 :257–262. 10.1038/nn.2726 [ PubMed ] [ CrossRef ] [ Google Scholar ]
- Salimpoor VN, van den Bosch I, Kovacevic N, McIntosh AR, Dagher A, Zatorre RJ (2013) Interactions between the nucleus accumbens and auditory cortices predict music reward value . Science 340 :216–219. 10.1126/science.1231059 [ PubMed ] [ CrossRef ] [ Google Scholar ]
- Särkämö T, Tervaniemi M, Laitinen S, Forsblom A, Soinila S, Mikkonen M, Autti T, Silvennoinen HM, Erkkilä J, Laine M, Peretz I, Hietanen M (2008) Music listening enhances cognitive recovery and mood after middle cerebral artery stroke . Brain 131 :866–876. 10.1093/brain/awn013 [ PubMed ] [ CrossRef ] [ Google Scholar ]
- Savage PE, Brown S, Sakai E, Currie TE (2015) Statistical universals reveal the structures and functions of human music . Proc Natl Acad Sci USA 112 :8987–8992. 10.1073/pnas.1414495112 [ PMC free article ] [ PubMed ] [ CrossRef ] [ Google Scholar ]
- Schiavo JK, Valtcheva S, Bair-Marshall CJ, Song SC, Martin KA, Froemke RC (2020) Innate and plastic mechanisms for maternal behaviour in auditory cortex . Nature 587 :426–431. 10.1038/s41586-020-2807-6 [ PMC free article ] [ PubMed ] [ CrossRef ] [ Google Scholar ]
- Schlaug G, Marchina S, Norton A (2009) Evidence for plasticity in white-matter tracts of patients with chronic Broca's aphasia undergoing intense intonation-based speech therapy . Ann NY Acad Sci 1169 :385–394. 10.1111/j.1749-6632.2009.04587.x [ PMC free article ] [ PubMed ] [ CrossRef ] [ Google Scholar ]
- Schoneich S (2020) Neuroethology of acoustic communication in field crickets: from signal generation to song recognition in an insect brain . Prog Neurobiol 194 :101882. [ PubMed ] [ Google Scholar ]
- Schubotz RI (2007) Prediction of external events with our motor system: towards a new framework . Trends Cogn Sci 11 :211–218. 10.1016/j.tics.2007.02.006 [ PubMed ] [ CrossRef ] [ Google Scholar ]
- Schultz W (2016) Dopamine reward prediction-error signalling: a two-component response . Nat Rev Neurosci 17 :183–195. 10.1038/nrn.2015.26 [ PMC free article ] [ PubMed ] [ CrossRef ] [ Google Scholar ]
- Shany O, Singer N, Gold BP, Jacoby N, Tarrasch R, Hendler T, Granot R (2019) Surprise-related activation in the nucleus accumbens interacts with music-induced pleasantness . Soc Cogn Affect Neurosci 14 :459–470. 10.1093/scan/nsz019 [ PMC free article ] [ PubMed ] [ CrossRef ] [ Google Scholar ]
- Sihvonen AJ, Särkämö T, Leo V, Tervaniemi M, Altenmüller E, Soinila S (2017) Music-based interventions in neurological rehabilitation . Lancet Neurol 16 :648–660. 10.1016/S1474-4422(17)30168-0 [ PubMed ] [ CrossRef ] [ Google Scholar ]
- Skov M, Nadal M (2020) A farewell to art: aesthetics as a topic in psychology and neuroscience . Perspect Psychol Sci 15 :630–642. 10.1177/1745691619897963 [ PubMed ] [ CrossRef ] [ Google Scholar ]
- Strafella AP, Paus T, Barrett J, Dagher A (2001) Repetitive transcranial magnetic stimulation of the human prefrontal cortex induces dopamine release in the caudate nucleus . J Neurosci 21 :RC157. 10.1523/JNEUROSCI.21-15-j0003.2001 [ PMC free article ] [ PubMed ] [ CrossRef ] [ Google Scholar ]
- Stupacher J, Hove MJ, Novembre G, Schutz-Bosbach S, Keller PE (2013) Musical groove modulates motor cortex excitability: aTMS investigation . Brain Cogn 82 :127–136. 10.1016/j.bandc.2013.03.003 [ PubMed ] [ CrossRef ] [ Google Scholar ]
- Swarbrick D, Bosnyak D, Livingstone SR, Bansal J, Marsh-Rollo S, Woolhouse MH, Trainor LJ (2018) How live music moves us: head movement differences in audiences to live versus recorded music . Front Psychol 9 :2682. 10.3389/fpsyg.2018.02682 [ PMC free article ] [ PubMed ] [ CrossRef ] [ Google Scholar ]
- Teki S, Grube M, Kumar S, Griffiths TD (2011) Distinct neural substrates of duration-based and beat-based auditory timing . J Neurosci 31 :3805–3812. 10.1523/JNEUROSCI.5561-10.2011 [ PMC free article ] [ PubMed ] [ CrossRef ] [ Google Scholar ]
- Trainor LJ, Cirelli L (2015) Rhythm and interpersonal synchrony in early social development . Ann NY Acad Sci 1337 :45–52. 10.1111/nyas.12649 [ PubMed ] [ CrossRef ] [ Google Scholar ]
- Turner C, Visentin P, Oye D, Rathwell S, Shan G (2021) Pursuing artful movement science in music performance: single subject motor analysis with two elite pianists . Percept Mot Skills 128 :1252–1274. 10.1177/00315125211003493 [ PMC free article ] [ PubMed ] [ CrossRef ] [ Google Scholar ]
- Varlet M, Nozaradan S, Nijhuis P, Keller PE (2020) Neural tracking and integration of 'self' and 'other' in improvised interpersonal coordination . Neuroimage 206 :116303. 10.1016/j.neuroimage.2019.116303 [ PubMed ] [ CrossRef ] [ Google Scholar ]
- Vuust P, Heggli OA, Friston KJ, Kringelbach ML (2022) Music in the brain . Nat Rev Neurosci 23 :287–305. 10.1038/s41583-022-00578-5 [ PubMed ] [ CrossRef ] [ Google Scholar ]
- Wang Y, Brzozowska-Prechtl A, Karten HJ (2010) Laminar and columnar auditory cortex in avian brain . Proc Natl Acad Sci USA 107 :12676–12681. 10.1073/pnas.1006645107 [ PMC free article ] [ PubMed ] [ CrossRef ] [ Google Scholar ]
- Wheeler BL (ed) (2015) Music therapy handbook: creative arts and play therapy . New York: Guildford. [ Google Scholar ]
- Witek MA, Clarke EF, Wallentin M, Kringelbach ML, Vuust P (2014) Syncopation, body-movement and pleasure in groove music . PLoS One 9 :e94446. 10.1371/journal.pone.0094446 [ PMC free article ] [ PubMed ] [ CrossRef ] [ Google Scholar ]
- Zatorre RJ (2023) From perception to pleasure: the neuroscience of music and why we love it . New York: Oxford UP. [ Google Scholar ]
- Zatorre RJ, Chen JL, Penhune VB (2007) When the brain plays music: auditory–motor interactions in music perception and production . Nat Rev Neurosci 8 :547–558. 10.1038/nrn2152 [ PubMed ] [ CrossRef ] [ Google Scholar ]
- Zhou W, Ye C, Wang H, Mao Y, Zhang W, Liu A, Yang CL, Li T, Hayashi L, Zhao W, Chen L, Liu Y, Tao W, Zhang Z (2022) Sound induces analgesia through corticothalamic circuits . Science 377 :198–204. 10.1126/science.abn4663 [ PMC free article ] [ PubMed ] [ CrossRef ] [ Google Scholar ]
- Zumbansen A, Peretz I, Hebert S (2014) The combination of rhythm and pitch can account for the beneficial effect of melodic intonation therapy on connected speech improvements in Broca's aphasia . Front Hum Neurosci 8 :592. [ PMC free article ] [ PubMed ] [ Google Scholar ]
Maribou State cancel shows as Chris Davids issues statement on rare brain condition
"We’re incredibly frustrated but touring requires so much more love and energy than I’m currently able to give"

Maribou State have announced that they are cancelling all upcoming live shows, while Chris Davids continues to recover following brain surgery.
The electronic duo have also confirmed that they have decided to postpone the release of their third studio album, as the artist allows himself time to return to full strength.
They had been due to make a number of festival appearances over the summer, including at Stowaway Festival in Buckinghamshire in August, followed by Lost Village in Lincolnshire , and Forwards Bristol to round out the month.
But as confirmed in a personal message on Instagram, they are putting all official public engagements on hold.
“Over the past few years Liam and I have hidden ourselves away to write our third album, a process which has not been an easy one,” Davids wrote. “In 2022 I was diagnosed with a rare brain condition called Chiari malformation. It had been the cause of a mix of symptoms including; debilitating headaches, insomnia and bad mental health, which have been hugely impacting my life and productivity in the studio.”
“After trying a variety of conservative treatments, I made the difficult decision to undergo brain surgery in November last year. The surgery, for the most part, was successful and the symptoms have now either been resolved or are in remission, however there were multiple complications that have since slowed down my recovery and presented me with new challenges, that five months on, are still ongoing.”
View this post on Instagram A post shared by Maribou State (@mariboustate)
Recommended
“As a result, we’ve had to make the hard decision of pressing pause on the project for the time being. We are therefore very sad to say we are cancelling all our live shows this year and postponing the release of any new music.”
“We know this will come as very disappointing news for those who have purchased tickets and have been patiently waiting on new music. We’re incredibly frustrated but touring requires so much more love and energy than I’m currently able to give. We hope you understand our reasoning for making this decision.”
He continued: “On a more positive note, I AM making progress, Liam [Ivory] IS in good health and able to continue DJ’ing, we DO have a finished third album; one we’re both incredibly proud of and excited to share with you all, and the live band WILL be getting back together as soon as the albums released.”
“Thank you all so much for your patience. It’s been a long journey since the last album but we’re both so grateful for the all the love and support you’ve continued to show, and we both truly can’t wait to send some of that back your way once I’m out the other side. Love, Chris x”
Maribou State’s last full-length studio album was 2018’s ‘Kingdoms In Colour’, which NME gave a four-star review . “Take a deep breath, lay back and soak in the technicolour empire Maribou State have crafted on this album – you’ll feel at one with our world for it,” concluded the review.
- Related Topics
You May Also Like
‘back to black’ review: marisa abela makes a worthy amy winehouse, kanii: “i don’t hold back when i express how i feel”, ‘fallout’ review: learn to love the bomb in this fun yet flawed adaptation, grief empowered actor abubakar salim – now he’s making a video game about it, more stories, amy winehouse’s ex-husband blake fielder-civil reacts to new film ‘back to black’, nick cave and warren ellis share ‘song for amy’ from new amy winehouse film ‘back to black’, palace talk “ferocious, beautiful” new album ‘ultrasound’: “there is often great hope to be found in difficult times”, glastonbury 2024 adds more names to line-up as stage announcements begin with acoustic stage, john squire says the stone roses “aren’t sending each other christmas cards or anything”.
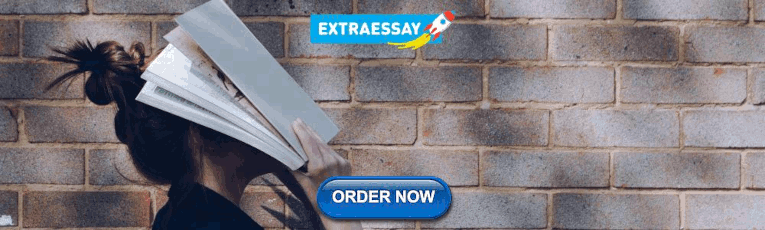
IMAGES
VIDEO
COMMENTS
These findings demonstrate bottom-up and top-down processes in the cerebral cortex during music listening and recall and provide important insights into music processing by the human brain. SIGNIFICANCE STATEMENT Understanding how the brain analyzes, stores, and retrieves music remains one of the most challenging problems in neuroscience.
Music, Brain Plasticity, Music Therapy, Cognition and Memory 1. Introduction With the rapid development of cognitive neuroscience and neuroimaging tech-nology, there are more and more researches focused on exploring the relation-ship between music and its effects on the brain. Since music is fundamentally How to cite this paper: Zhang, S.Q. (2020)
Abstract. Through music we can learn much about our human origins and the human brain. Music is a potential method of therapy and a means of accessing and stimulating specific cerebral circuits. There is also an association between musical creativity and psychopathology. This paper provides a brief review.
Abstract. Music is ubiquitous across human cultures — as a source of affective and pleasurable experience, moving us both physically and emotionally — and learning to play music shapes both ...
We, the thesis committee for the above candidate for the Master of Science degree, hereby recommend acceptance of this thesis. Kathleen Murphy, PhD, LCAT, MT-BC ... rapid review includes forty studies that provide neural evidence for the effects of music on anxiety-related brain structures, specifically the amygdala, hippocampus, and insula. The
Donald A. Hodges (2000) summarizes important findings from the study of music and cerebral activity, and states the following premises: 1) the human brain responds to and participates in music; 2 ...
Abstract. Through music we can learn much about our human origins and the human brain. Music is a potential method of therapy and a means of accessing and stimulating specific cerebral circuits ...
The brain function on music has been a recently developing field of neuroscience that holds a position of great interest to neuroscientists, psychologists, health professionals, and musicians alike. The primary goal of investigating music and the brain has been to determine particular neural correlates that are involved in or altered by the ...
Abstract and Figures. The final purpose of this thesis is to explain and justify how early music training can be considered an additional educational tool to prepare the brain for a better ...
Music, as Thiam (2006, p. 97) notes, has the power of healing our frequent ailments as human beings. In addition, research validates that the classical forms of music has had an impact on individuals, reporting impressive results on the power of healing particularly (Effects of Music on the Mind and Brain 2014).Music has a calming effect on the human mind, and an inspiring zeal on the general ...
brain development for the incorporation of music. One of the differences between the developed . brains of . Homo sapiens . and those of the great apes is the increase in area allocated to processing auditory information. Thus, in other primates the size of the visual cortex correlates well with brain size, but in . Homo sapiens. it is smaller ...
Music of varied kinds consistently triggers a large range of drives and emotions, which, in turn, induce a particular class of mental experiences known as feelings. The feelings are often pleasurable, though not necessarily. Neuroimaging and electrophysiological studies, in normal individuals as well as in patients with focal neurological lesions, reveal that music can change the state of ...
The term music refers to structured sounds that are produced by humans as a means of social interaction, expression, diversion, or evocation of emotion. Making music in a group is a tremendously demanding task for the human brain, and it elicits a large array of cognitive (and affective) processes, including perception, multimodal integration, attention, social cognition, memory, and ...
The following are a just a sample of the amazing ways music affects the brain: 1) Music can strengthen our bonds with others. A study by Florida International University's Community Based Research Institute has found that ensemble based musical instruction for young children (ages 8-17) had significant increases on kid's competence ...
This thesis will explore the research on implementing music therapy into the care of ... Statement of Purpose ... music and language processing structures in the brain, which might explain why music therapy can be beneficial when addressing neurodegenerative symptoms (Zhang, Cai, An, Hui, Ren, Ma, ...
Cognitive, emotional and social functions in music perception and production. Listening to music requires certain perceptual abilities, including pitch discrimination, auditory memory, and selective attention in order to perceive the temporal and harmonic structure of the music as well as its affective components, and engages a distributed network of brain structures (Peretz and Zatorre, 2005).
brain regions associated with reward from drug addiction and food consumption (Weinberger, 2004). While there have been many studies on music portraying how the emotional experience with music is a multifaceted phenomenon, the present study examined emotion regulation, music and how it relates to psychological well-being. Music
This paper will discuss, in short, how music influences our brain, as well as some behavioral and physiological effects as a result. The brain consists of many regions responsible for different cognitive processes, such as learning, memory, recall, speech, and our emotions. It has been found that music helps to facilitate all of these cognitive processes, regardless of its emotional valence or ...
helps people with Parkinson' s disease who's ability to walk has been impacted to walk. easier and faster. This essay's goal is to explore this deep interconnection between certain brain ...
A thesis statement is not a statement of fact. It is an assertive statement that states your claims and that you can prove with evidence. It should be the product of research and your own critical thinking. There are different ways and different approaches to write a thesis statement. Here are some steps you can try to create a thesis statement: 1.
Thesis Statement About Music and the Brain - Free download as PDF File (.pdf), Text File (.txt) or read online for free. thesis statement about music and the brain
NEW YORK (AP) — Maggie Rogers wrote and recorded her new album, "Don't Forget Me," over five days last winter. The songs came quickly and chronologically, as if she was writing "different scenes in a movie." "I've been writing songs now for 15 years, and so I think I've just come into this place where I really trust my process and my craft.
SIGNIFICANCE STATEMENT Music and music-based interventions (MBI) engage a wide range of brain circuits and hold promising therapeutic potentials for a variety of health conditions. Comparative studies using animal models have helped in uncovering brain circuit activities involved in rhythm perception, while human imaging, brain stimulation, and ...
Maribou State have announced that they are cancelling all upcoming live shows, while Chris Davids continues to recover following brain surgery. The electronic duo have also confirmed that they ...