Thank you for visiting nature.com. You are using a browser version with limited support for CSS. To obtain the best experience, we recommend you use a more up to date browser (or turn off compatibility mode in Internet Explorer). In the meantime, to ensure continued support, we are displaying the site without styles and JavaScript.
- View all journals
- My Account Login
- Explore content
- About the journal
- Publish with us
- Sign up for alerts
- Open access
- Published: 03 August 2022
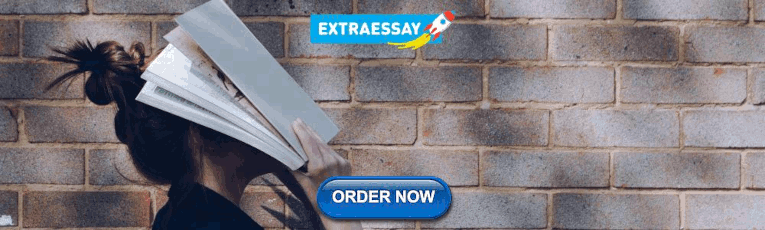
The challenge of unprecedented floods and droughts in risk management
- Heidi Kreibich ORCID: orcid.org/0000-0001-6274-3625 1 ,
- Anne F. Van Loon ORCID: orcid.org/0000-0003-2308-0392 2 ,
- Kai Schröter ORCID: orcid.org/0000-0002-3173-7019 1 , 3 ,
- Philip J. Ward ORCID: orcid.org/0000-0001-7702-7859 2 ,
- Maurizio Mazzoleni ORCID: orcid.org/0000-0002-0913-9370 2 ,
- Nivedita Sairam ORCID: orcid.org/0000-0003-4611-9894 1 ,
- Guta Wakbulcho Abeshu ORCID: orcid.org/0000-0001-8775-3678 4 ,
- Svetlana Agafonova ORCID: orcid.org/0000-0002-6392-1662 5 ,
- Amir AghaKouchak ORCID: orcid.org/0000-0003-4689-8357 6 ,
- Hafzullah Aksoy ORCID: orcid.org/0000-0001-5807-5660 7 ,
- Camila Alvarez-Garreton ORCID: orcid.org/0000-0002-5381-4863 8 , 9 ,
- Blanca Aznar ORCID: orcid.org/0000-0001-6952-0790 10 ,
- Laila Balkhi ORCID: orcid.org/0000-0001-8224-3556 11 ,
- Marlies H. Barendrecht ORCID: orcid.org/0000-0002-3825-0123 2 ,
- Sylvain Biancamaria ORCID: orcid.org/0000-0002-6162-0436 12 ,
- Liduin Bos-Burgering ORCID: orcid.org/0000-0002-8372-4519 13 ,
- Chris Bradley ORCID: orcid.org/0000-0003-4042-867X 14 ,
- Yus Budiyono ORCID: orcid.org/0000-0002-6288-6527 15 ,
- Wouter Buytaert ORCID: orcid.org/0000-0001-6994-4454 16 ,
- Lucinda Capewell 14 ,
- Hayley Carlson 11 ,
- Yonca Cavus ORCID: orcid.org/0000-0002-0528-284X 17 , 18 , 19 ,
- Anaïs Couasnon ORCID: orcid.org/0000-0001-9372-841X 2 ,
- Gemma Coxon ORCID: orcid.org/0000-0002-8837-460X 20 , 21 ,
- Ioannis Daliakopoulos ORCID: orcid.org/0000-0001-9333-4963 22 ,
- Marleen C. de Ruiter ORCID: orcid.org/0000-0001-5991-8842 2 ,
- Claire Delus ORCID: orcid.org/0000-0002-6690-5326 23 ,
- Mathilde Erfurt ORCID: orcid.org/0000-0003-1389-451X 19 ,
- Giuseppe Esposito ORCID: orcid.org/0000-0001-5638-657X 24 ,
- Didier François 23 ,
- Frédéric Frappart ORCID: orcid.org/0000-0002-4661-8274 25 ,
- Jim Freer 20 , 21 , 26 ,
- Natalia Frolova ORCID: orcid.org/0000-0003-3576-285X 5 ,
- Animesh K. Gain ORCID: orcid.org/0000-0003-3814-693X 27 , 28 ,
- Manolis Grillakis ORCID: orcid.org/0000-0002-4228-1803 29 ,
- Jordi Oriol Grima 10 ,
- Diego A. Guzmán 30 ,
- Laurie S. Huning ORCID: orcid.org/0000-0002-0296-4255 6 , 31 ,
- Monica Ionita ORCID: orcid.org/0000-0001-8240-4380 32 , 33 , 34 ,
- Maxim Kharlamov ORCID: orcid.org/0000-0002-4439-5193 5 , 35 ,
- Dao Nguyen Khoi ORCID: orcid.org/0000-0002-1618-1948 36 ,
- Natalie Kieboom ORCID: orcid.org/0000-0001-8497-0204 37 ,
- Maria Kireeva ORCID: orcid.org/0000-0002-8285-9761 5 ,
- Aristeidis Koutroulis ORCID: orcid.org/0000-0002-2999-7575 38 ,
- Waldo Lavado-Casimiro ORCID: orcid.org/0000-0002-0051-0743 39 ,
- Hong-Yi Li ORCID: orcid.org/0000-0002-9807-3851 4 ,
- María Carmen LLasat ORCID: orcid.org/0000-0001-8720-4193 40 , 41 ,
- David Macdonald ORCID: orcid.org/0000-0003-3475-636X 42 ,
- Johanna Mård ORCID: orcid.org/0000-0002-8789-7628 43 , 44 ,
- Hannah Mathew-Richards 37 ,
- Andrew McKenzie ORCID: orcid.org/0000-0001-8723-4325 42 ,
- Alfonso Mejia ORCID: orcid.org/0000-0003-3891-1822 45 ,
- Eduardo Mario Mendiondo ORCID: orcid.org/0000-0003-2319-2773 46 ,
- Marjolein Mens 47 ,
- Shifteh Mobini ORCID: orcid.org/0000-0002-3365-7346 48 , 49 ,
- Guilherme Samprogna Mohor ORCID: orcid.org/0000-0003-2348-6181 50 ,
- Viorica Nagavciuc ORCID: orcid.org/0000-0003-1111-9616 32 , 34 ,
- Thanh Ngo-Duc ORCID: orcid.org/0000-0003-1444-7498 51 ,
- Thi Thao Nguyen Huynh ORCID: orcid.org/0000-0001-9071-1225 52 ,
- Pham Thi Thao Nhi ORCID: orcid.org/0000-0003-4118-8479 36 ,
- Olga Petrucci ORCID: orcid.org/0000-0001-6918-1135 24 ,
- Hong Quan Nguyen 52 , 53 ,
- Pere Quintana-Seguí ORCID: orcid.org/0000-0002-7107-9671 54 ,
- Saman Razavi ORCID: orcid.org/0000-0003-1870-5810 11 , 55 , 56 ,
- Elena Ridolfi ORCID: orcid.org/0000-0002-4714-2511 57 ,
- Jannik Riegel 58 ,
- Md Shibly Sadik ORCID: orcid.org/0000-0001-9205-4791 59 ,
- Elisa Savelli ORCID: orcid.org/0000-0002-8948-0316 43 , 44 ,
- Alexey Sazonov 5 , 35 ,
- Sanjib Sharma ORCID: orcid.org/0000-0003-2735-1241 60 ,
- Johanna Sörensen ORCID: orcid.org/0000-0002-2312-4917 49 ,
- Felipe Augusto Arguello Souza ORCID: orcid.org/0000-0002-2753-9896 46 ,
- Kerstin Stahl ORCID: orcid.org/0000-0002-2159-9441 19 ,
- Max Steinhausen ORCID: orcid.org/0000-0002-8692-8824 1 ,
- Michael Stoelzle ORCID: orcid.org/0000-0003-0021-4351 19 ,
- Wiwiana Szalińska ORCID: orcid.org/0000-0001-6828-6963 61 ,
- Qiuhong Tang 62 ,
- Fuqiang Tian ORCID: orcid.org/0000-0001-9414-7019 63 ,
- Tamara Tokarczyk ORCID: orcid.org/0000-0001-5862-6338 61 ,
- Carolina Tovar ORCID: orcid.org/0000-0002-8256-9174 64 ,
- Thi Van Thu Tran ORCID: orcid.org/0000-0003-1187-3520 52 ,
- Marjolein H. J. Van Huijgevoort ORCID: orcid.org/0000-0002-9781-6852 65 ,
- Michelle T. H. van Vliet ORCID: orcid.org/0000-0002-2597-8422 66 ,
- Sergiy Vorogushyn ORCID: orcid.org/0000-0003-4639-7982 1 ,
- Thorsten Wagener ORCID: orcid.org/0000-0003-3881-5849 21 , 50 , 67 ,
- Yueling Wang 62 ,
- Doris E. Wendt ORCID: orcid.org/0000-0003-2315-7871 67 ,
- Elliot Wickham 68 ,
- Long Yang ORCID: orcid.org/0000-0002-1872-0175 69 ,
- Mauricio Zambrano-Bigiarini ORCID: orcid.org/0000-0002-9536-643X 8 , 9 ,
- Günter Blöschl ORCID: orcid.org/0000-0003-2227-8225 70 &
- Giuliano Di Baldassarre ORCID: orcid.org/0000-0002-8180-4996 43 , 44 , 71
Nature volume 608 , pages 80–86 ( 2022 ) Cite this article
53k Accesses
128 Citations
962 Altmetric
Metrics details
- Natural hazards
Risk management has reduced vulnerability to floods and droughts globally 1 , 2 , yet their impacts are still increasing 3 . An improved understanding of the causes of changing impacts is therefore needed, but has been hampered by a lack of empirical data 4 , 5 . On the basis of a global dataset of 45 pairs of events that occurred within the same area, we show that risk management generally reduces the impacts of floods and droughts but faces difficulties in reducing the impacts of unprecedented events of a magnitude not previously experienced. If the second event was much more hazardous than the first, its impact was almost always higher. This is because management was not designed to deal with such extreme events: for example, they exceeded the design levels of levees and reservoirs. In two success stories, the impact of the second, more hazardous, event was lower, as a result of improved risk management governance and high investment in integrated management. The observed difficulty of managing unprecedented events is alarming, given that more extreme hydrological events are projected owing to climate change 3 .
Similar content being viewed by others
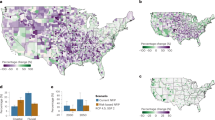
How the USA can benefit from risk-based premiums combined with flood protection
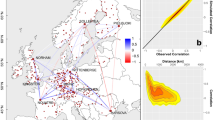
Biases in national and continental flood risk assessments by ignoring spatial dependence
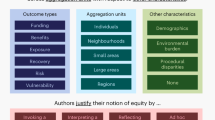
Developing more useful equity measurements for flood-risk management
Observed decreasing trends in the vulnerability to floods and droughts, owing to effective risk management, are encouraging 1 . Globally, human and economic vulnerability dropped by approximately 6.5- and 5-fold, respectively, between the periods 1980–1989 and 2007–2016 (ref. 2 ). However, the impacts of floods and droughts are still severe and increasing in many parts of the world 6 . Climate change will probably lead to a further increase in their impacts owing to projected increases in the frequency and severity of floods and droughts 3 . The economic damage of floods is projected to double globally 7 and that of droughts to triple in Europe 8 , for a mean temperature increase of 2 °C.
The purpose of risk management is to reduce the impact of events through modification of the hazard, exposure and/or vulnerability: according to United Nations (UN) terminology 9 , disaster risk management is the application of disaster risk reduction policies and strategies to prevent new disaster risk, reduce existing disaster risk and manage residual risk, contributing to the strengthening of resilience against, and reduction of, disaster losses. Hazard is a process, phenomenon or human activity that may cause loss of life, injury or other health impacts, property damage, social and economic disruption or environmental degradation; exposure is the situation of people, infrastructure, housing, production capacities and other tangible human assets located in hazard-prone areas; and vulnerability is the conditions determined by physical, social, economic and environmental factors or processes 10 , 11 , 12 , 13 that increase the susceptibility of an individual, a community, assets or systems to the impacts of hazards. To be effective, risk management needs to be based on a sound understanding of these controlling risk drivers 14 , 15 . Past studies have identified increasing exposure as a primary driver of increasing impacts 3 , 4 , and vulnerability reduction has been identified as key for reduction of impacts 16 , 17 . However, ascertaining the combined effect of the drivers and the overall effectiveness of risk management has been hampered by a lack of empirical data 4 , 5 .
Here we analyse a new dataset of 45 pairs of flood or drought events that occurred in the same area on average 16 years apart (hereinafter referred to as paired events). The data comprise 26 flood and 19 drought paired events across different socioeconomic and hydroclimatic contexts from all continents (Fig. 1a ). We analyse floods and droughts together, because of the similarity of some of the management methods (for example, warning systems, water reservoir infrastructure), the potential for trade-offs in risk reduction between floods and droughts and therefore value for the management communities to learn from each other 18 . The impact, quantified by direct (fatalities, monetary damage), indirect (for example, disruption of traffic or tourism) and intangible impacts (for example, impact on human health or cultural heritage), is considered to be controlled by three drivers: hazard, exposure and vulnerability 3 . These drivers are quantified using a large range of different indices—for example, the standardized precipitation index, the number of houses in the affected area and risk awareness, respectively (Supplementary Table 1 ). These three drivers are considered to be exacerbated by management shortcomings. Hazard may be exacerbated by problems with water management infrastructure such as levees or reservoirs 19 . Exposure and vulnerability may be worsened by suboptimal implementation of non-structural measures such as risk-aware regional planning 20 or early warning 21 , respectively. We analyse management shortcomings and their effect on the three drivers explicitly, as this is the point at which improvements can start—for example, by the introduction of better strategies and policies. Data availability understandably varies among the paired events, and this can introduce inconsistency and subjectivity. The analyses are therefore based on indicators of change, to account for differences between paired events in respect of measured variables, data quality and uncertainty. These indicators of change represent the differences between the first event (baseline) and the second, categorized as large decreases/increases (−2/+2), small decreases/increases (−1/+1) and no change (0) (Supplementary Table 2 ). To minimize the subjectivity and uncertainty of indicator assignment, a quality assurance protocol is implemented and indicators of change with sub-indicators are used.
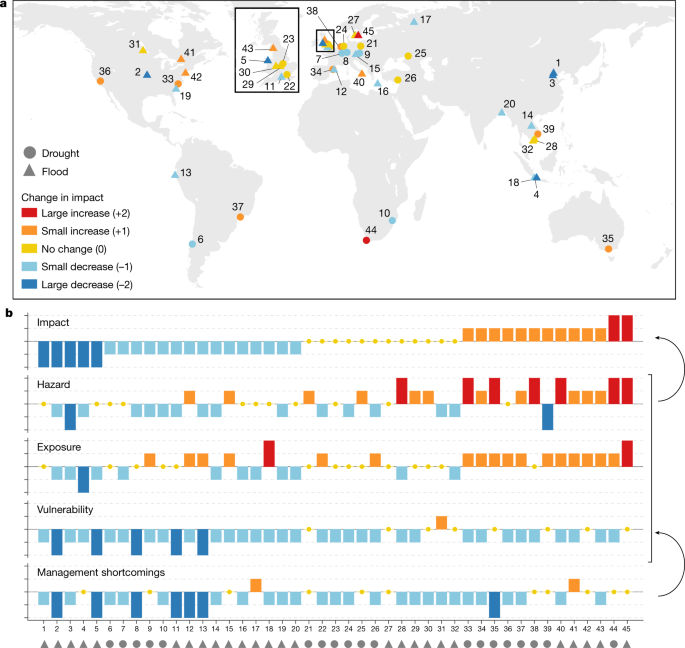
a , Location of flood and drought paired events ( n = 45). Numbers are paired-event IDs. b , Indicators of change, sorted by impact change. Impact is considered to be controlled by hazard, exposure and vulnerability, which are exacerbated by risk management shortcomings. Maps of the paired events coloured according to drivers and management shortcomings are shown in Extended Data Fig. 1 .
Source data
The majority of paired events show decreases in management shortcomings (71% of paired events; Fig. 1b ), which reflects that societies tend to learn from extreme events 22 . Most cases also show a decrease in vulnerability (80% of paired events) as societies typically reduce their vulnerability after the first event of a pair 21 . The five paired events with a large decrease in impact (dark blue, top left in Fig. 1b ) are associated with decreases or no change of all three drivers.
Drivers of changes in impact
Changes in flood impacts are significantly and positively correlated with changes in hazard ( r = 0.64, P ≤ 0.01), exposure ( r = 0.55, P ≤ 0.01) and vulnerability ( r = 0.60, P ≤ 0.01) (Fig. 2a ), which is in line with risk theory 3 . Although a previous analysis of eight case studies 21 identified vulnerability as a key to reduction of flood impacts, this new, more comprehensive, dataset suggests that changes in hazard, exposure and vulnerability are equally important, given that they correlate equally strongly with changes in flood impact. Changes in drought impacts are significantly correlated with changes in hazard and exposure, but not with changes in vulnerability (Fig. 2c ). This suggests that changes in vulnerability have been less successful in reducing drought impact than flood impact, which is also consistent with those event pairs for which only vulnerability changed (Extended Data Table 1 ). However, quantification of the contribution of individual drivers is difficult with this empirical approach because there are only a limited number of cases in which only one driver changed. There are three cases in which only vulnerability changed between events, two cases in which only hazard changed and no case in which only exposure changed (Extended Data Table 1 ). Additionally, paired events without a change in hazard (0) are analysed in more detail to better understand the role of exposure and vulnerability (Extended Data Fig. 2 ). In all these paired events, a reduction in impact was associated with a reduction in vulnerability, highlighting the importance of vulnerability. In five of these eight cases with a decrease in impact there was also a decrease in exposure, whereas in one case (floods in Jakarta, Indonesia in 2002 and 2007 (ID 18)) there was a large increase in exposure. In the paired event of droughts in California, United States (1987–1992 and 2011–2016, ID 36) an increase in exposure and a reduction in vulnerability increased impact, which points to the more important role of exposure in comparison with vulnerability in this drought case (Extended Data Fig. 2 ).
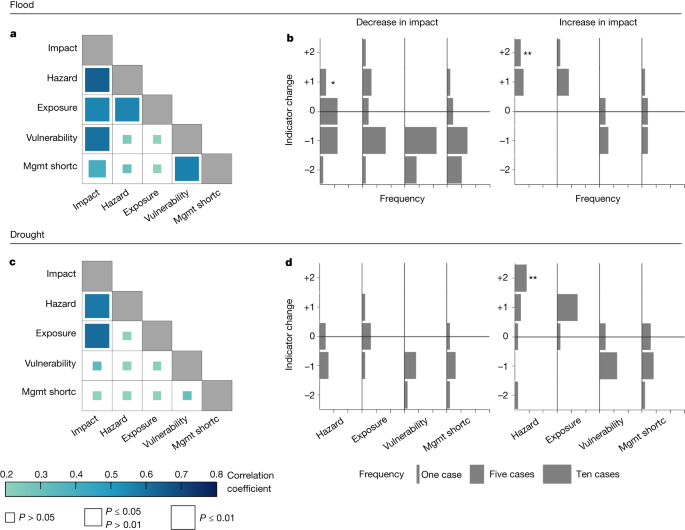
a , c , Correlation matrix of indicators of change for flood ( a ) and drought ( c ) paired events. Colours of squares indicate Spearman’s rank correlation coefficients and their size, the P value. b , d ,Histograms of indicators of change of flood ( b ) and drought ( d ) stratified by decrease ( n = 15 and n = 5 paired events for flood and drought, respectively) and increase ( n = 5 and n = 8 paired events, respectively) in impact. The asterisk denotes the success stories of Box 1 ; double asterisks denote pairs for which the second event was much more hazardous than the first (that is, 'unprecedented'). Mgmt shortc, management shortcomings.
Generally the changes in drivers are not significantly correlated with each other, with the exception of hazard and exposure in the case of floods ( r = 0.55, P ≤ 0.01) (Fig. 2a ). This finding may be explained by the influence of hazard on the size of the inundation area, and thus on the numbers of people and assets affected, which represent exposure.
The sensitivity analysis suggests that the correlation pattern is robust, as visualized by the colours in Extended Data Fig. 3 . The pattern of P values is also robust for flood cases, although these become less significant for drought because of the smaller sample size (Extended Data Fig. 3 ).
We split the paired events into groups of decreasing and increasing impact to evaluate their drivers separately (Fig. 2b,d ). Overall, the pattern is similar for floods and droughts. Most flood and drought pairs with decreasing impact show either a decrease in hazard (ten pairs, 50%) or no change (eight pairs, 40%). Exceptions are two flood pairs that are success stories of decreased impact despite an increase in hazard, as detailed in Box 1 . The change in exposure of the pairs with decreased impacts (Fig. 2b,d ) ranges from a large decrease to a large increase, whereas vulnerability always decreased. All cases with a large decrease in vulnerability (−2) are associated with a decrease in impacts. Overall, the pattern suggests that a decrease in impacts is mainly caused by a combination of lower hazard and vulnerability, despite an increase in exposure in 25% of cases.
The role of hazard and vulnerability in impact reduction can be exemplified by the pair of riverine floods in Jakarta, Indonesia (ID 4 in Fig. 1 ). The 2007 event had a flood return period of 50 years, whereas it was 30 years for the 2013 event 23 (that is, the hazard of the second event was smaller). Vulnerability had also decreased as a result of improved preparedness resulting from a flood risk mapping initiative and capacity building programmes implemented after the first flood, to improve citizens' emergency response, as well as by an improvement in official emergency management by establishment of the National Disaster Management Agency in 2008. Additionally, exposure was substantially reduced. Whilst the first flood caused 79 fatalities and direct damage of €1.3 billion, the second event caused 38 fatalities and €0.76 billion of direct damage.
Another example is a pair of Central European droughts (ID 9). During the 2003 event, the minimum 3-month Standardized Precipitation Evapotranspiration Index was −1.62 whereas in 2015 it was −1.18—that is, the hazard of the second event was smaller 24 . The vulnerability was also lower in the second event, because the first event had raised public awareness and triggered an improvement in institutional planning. For instance, the European Commission technical guidance on drought management plans 25 was implemented. Many reservoirs were kept filled until the beginning of summer 2015, which alleviated water shortages for various sectors and, in some cities (for example, Bratislava and Bucharest), water was supplied from tanks 26 . Additionally, water use and abstraction restrictions were implemented for non-priority uses including irrigation 26 . The impact was reduced from €17.1 billion to €2.2 billion, despite an increase in exposure because of the larger drought extent affecting almost all of Europe in 2013.
Most flood and drought pairs with an increase in impact also show a larger hazard (11 cases, 85%; Fig. 2b,d ). For six of these paired events (46%), the second event was much more hazardous than the first (hazard indicator-of-change +2), whereas this was never the case for the pairs with decreasing impact. Of those pairs with an increase in impact, 12 (92%) show an increase in exposure and nine (69%) show a small decrease in vulnerability (vulnerability indicator-of-change −1). Overall, the pattern suggests that the increase in impact is mainly caused by a combination of higher hazard and exposure, which is not compensated by a small decrease in vulnerability.
The role of hazard and exposure in increasing impact is illustrated by a pair of pluvial floods in Corigliano-Rossano City, Calabria, Italy (ID 40). This 2015 event was much more hazardous (+2) than that in 2000, with precipitation return periods of more than 100 and 10–20 years, respectively 27 . Also, the 2000 event occurred during the off-season for tourism in September whereas the exposure was much larger in 2015, because the event occurred in August when many tourists were present. Interruption of the peak holiday season caused severe indirect economic damage. Another example is a pair of droughts (ID 33) affecting North Carolina, United States. Between 2007 and 2009, about 65% of the state was affected by what was classified as an exceptional drought, with a composite drought indicator of the US Drought Monitor of 27 months 28 , whereas between 2000 and 2003 only about 30% of the state was affected by an exceptional drought of 24 months 28 . The crop losses in 2007–2009 were about €535 million, whereas they were €497 million in 2000–2003, even though vulnerability had been reduced due to drought early warning and management by the North Carolina Drought Management Council, established in 2003.
Box 1 Success stories of decreased impact despite increased hazard
The dataset includes two cases in which a lower impact was achieved despite a larger hazard of the second event, making these interesting success stories (Fig. 3 ). Both cases are flood paired events, but of different types (that is, pluvial and riverine floods (Table 1 )). These cases have in common that institutional changes and improved flood risk management governance were introduced and high investments in integrated management were undertaken, which led to an effective implementation of structural and non-structural measures, such as improved early warning and emergency response to complement structural measures such as levees (Table 1 ).
Effects of changes in management on drivers
The correlations shown in Fig. 2a,c also shed light on how management affects hazard, exposure and vulnerability and thus, indirectly, impact. For flood paired events, changes in management shortcomings are significantly positively correlated with changes in vulnerability ( r = 0.56, P ≤ 0.01), and both are significantly positively correlated with changes in impact (Fig. 2a ). For drought, however, these correlations are not significant (Fig. 2c ). Thus, achieving decreases in vulnerability, and consequently in impact, by improving risk management (that is, reducing management shortcomings) seems to be more difficult for droughts than for floods. This difficulty may be related to spillover effects—that is, drought measures designed to reduce impacts in one sector can increase impacts in another. For example, irrigation to alleviate drought in agriculture may increase drought impacts on drinking water supply and ecology 29 .
The paired floods in the Piura region, Peru (ID 13) illustrate how effective management can reduce vulnerability, and consequently impact. At the Piura river, maximum flows of 3,367 and 2,755 m 3 s −1 were recorded during the 1998 and 2017 events, respectively (that is, hazard showed a small decrease (−1)). Around 2000, the national hydrometeorological service started to issue medium-range weather forecasts that allowed preparations months before the 2017 event. In 2011, the National Institute of Civil Defence and the National Centre for the Estimation, Prevention, and Reduction of Disaster Risk were founded which, together with newly established short-range river flow forecasts, allowed more efficient emergency management of the more recent event. Additionally, non-governmental organizations such as Practical Action had implemented disaster risk-reduction activities, including evacuation exercises and awareness campaigns 30 . All of these improvements in management decreased vulnerability. The impact of the second event was smaller, with 366 fatalities in 1998 compared with 159 in 2017, despite an increase in exposure due to urbanization and population increase.
When the hazard of the second event was larger than that of the first (+1, +2), in 11 out of 18 cases (61%) the impact of the second event was also larger, irrespective of small decreases in vulnerability in eight of these cases (light blue dots/triangles in Fig. 3 ). There are only two paired events in our dataset for which a decrease in impact was achieved despite the second event being more hazardous (highlighted by the green circle in Fig. 3 ). These cases are considered success stories and are further discussed in Box 1 . For the two paired events (ID 21 and 30) for which the only driver that changed was hazard (+1), the impacts did not change (0) (Extended Data Table 1 ). Water retention capacity of 189,881,000 m³ and good irrigation infrastructure with sprinkling machines were apparently sufficient to counteract the slight increase in hazard for the drought paired event in Poland in 2006 and 2015 (ID 21). The improved flood alleviation scheme implemented between the paired flood events (2016 and 2018), protected properties in Birmingham, United Kingdom (ID 30). There are, however, seven cases for which the second event was much more hazardous (+2) than the first (highlighted by the purple ellipse in Fig. 3 )—that is, events of a magnitude that locals had probably not previously experienced. We term these events, subjectively, as unprecedented; almost all had an increased impact despite improvements in management.
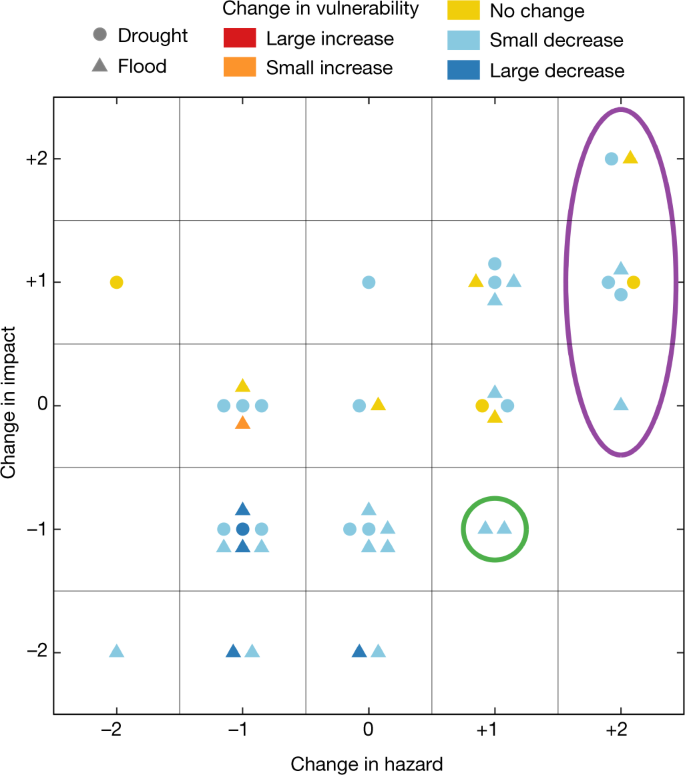
Categories are: lower hazard and lower impact, ten cases; higher hazard and higher impact, 11 cases; lower hazard and higher impact, one case; higher hazard and lower impact, two cases. Circles and triangles indicate drought and flood paired events, respectively; their colours indicate change in vulnerability. Green circle highlights success stories ( n = 2) of reduced impact (−1) despite a small increase in hazard (+1). Purple ellipse indicates paired events ( n = 7) with large increase in hazard (+2)—that is, events that were subjectively unprecedented and probably not previously experienced by local residents.
One unprecedented pluvial flood is the 2014 event in the city of Malmö, Sweden (ID 45). This event was much more hazardous than that experienced a few years before, with precipitation return periods on average of 135 and 24 years, respectively, for 6 h duration 31 . The largest 6 h precipitation measured at one of nine stations during the 2014 event corresponded to a return period of 300 years. The combined sewage system present in the more densely populated areas of the city was overwhelmed, leading to extensive basement flooding in 2014 (ref. 31 ). The direct monetary damage was about €66 million as opposed to €6 million in the first event. An unprecedented drought occurred in the Cape Town metropolitan area of South Africa, in 2015–2018 (ID 44). The drought was much longer (4 years) than that experienced previously in 2003–2004 (2 years). Although the Berg River Dam had been added to the city’s water supply system in 2009, and local authorities had developed various strategies for managing water demands (for example, water restrictions, tariff increases, communication campaign), the second event caused a much higher direct impact of about €180 million 32 because the water reserves were reduced to virtually zero.
Even though it is known that vulnerability reduction plays a key role in reducing risk, our paired-event cases reveal that when the hazard of the second event was higher than the first, a reduction in vulnerability alone was often not sufficient to reduce the impact of the second event to less than that of the first. Our analysis of drivers of impact change reveals the importance of reducing hazard, exposure and vulnerability to achieve an effective impact reduction (Fig. 2 ). Although previous studies have attributed a high priority to vulnerability reduction 17 , 21 , the importance of considering all three drivers identified here may reflect the sometimes limited efficiency of management decisions, resulting in unintended consequences. For example, levee construction aiming at reducing hazards may increase exposure through encouraging settlements in floodplains 33 , 34 . Similarly, construction of reservoirs to abate droughts may enhance exposure through encouraging agricultural development and thus increase water demand 35 , 36 .
Events that are much more hazardous than preceding events (termed unprecedented here) seem to be difficult to manage; in almost all the cases considered they led to increased impact (Fig. 3 ). This finding may be related to two factors. First, large infrastructure such as levees and water reservoirs play an important role in risk management. These structures usually have an upper design limit up to which they are effective but, once a threshold is exceeded, they become ineffective. For example, the unprecedented pluvial flood in 2014 in Malmö, Sweden (ID 45) exceeded the capacity of the sewer system 31 and the unprecedented drought in Cape Town (ID 44) exceeded the storage water capacity 37 . This means that infrastructure is effective in preventing damage during events of a previously experienced magnitude, but often fails for unprecedented events. Non-structural measures, such as risk-aware land-use planning, precautionary measures and early warning, can help mitigate the consequences of water infrastructure failure in such situations 21 , but a residual risk will always remain. Second, risk management is usually implemented after large floods and droughts, whereas proactive strategies are rare. Part of the reason for this behaviour is a cognitive bias associated with the rarity and uniqueness of extremes, and the nature of human risk perception, which makes people attach a large subjective probability to those events they have personally experienced 38 .
On the other hand, two case studies were identified in which impact was reduced despite an increase in hazard (Box 1 ). An analysis of these case studies identifies three success factors: (1) effective governance of risk and emergency management, including transnational collaboration such as in the Danube case; (2) high investments in structural and non-structural measures; and (3) improved early warning and real-time control systems such as in the Barcelona case. We believe there is potential for more universal application of these success factors to counteract the current trend of increasing impacts associated with climate change 3 . These factors may also be effective in the management of unprecedented events, provided they are implemented proactively.
The concept of paired events aims at comparing two events of the same hazard type that occurred in the same area 21 to learn from the differences and similarities. This concept is analogous to paired catchment studies, which compare two neighbouring catchments with different vegetation in terms of their water yield 39 . Our study follows the theoretical risk framework that considers impact as a result of three risk components or drivers 3 : hazard, exposure and vulnerability (Extended Data Fig. 4 ). Hazard reflects the intensity of an event, such as a flooded area or drought deficit—for example, measured by the standardized precipitation index. Exposure reflects the number of people and assets in the area affected by the event. Consequently, the change in exposure between events is influenced by changes in the population density and the assets in the affected area (socioeconomic developments), as well as by changes in the size of the affected area (change of hazard). Vulnerability is a complex concept, with an extensive literature from different disciplines on how to define, measure and quantify it 13 , 40 , 41 , 42 . For instance, Weichselgartner 43 lists more than 20 definitions of vulnerability, and frameworks differ quite substantially—for example, in terms of integration of exposure into vulnerability 11 or separating them 3 . Reviews and attempts to converge on the various vulnerability concepts stress that vulnerability is dynamic and that assessments should be conducted for defined human–environment systems at particular places 12 , 44 , 45 . Every vulnerability analysis requires an approach adapted to its specific objectives and scales 46 . The paired event approach allows detailed context and place-based vulnerability assessments that are presented in the paired event reports, as well as comparisons across paired events based on the indicators-of-change. The selection of sub-indicators for the characterization of vulnerability is undertaken with a particular focus on temporal changes at the same place. All three drivers—hazard, exposure and vulnerability—can be reduced by risk-management measures. Hazard can be reduced by structural measures such as levees or reservoirs 19 , exposure by risk-aware regional planning 20 and vulnerability by non-structural measures, such as early warning 21 .
Our comparative analysis is based on a novel dataset of 45 paired events from around the world, of which 26 event pairs are floods and 19 are droughts. The events occurred between 1947 and 2019, and the average period between the two events of a pair is 16 years. The number of paired events is sufficiently large to cover a broad range of hydroclimatic and socioeconomic settings around the world and allows differentiated, context-specific assessments on the basis of detailed in situ observations. Flood events include riverine, pluvial, groundwater and coastal floods 47 , 48 , 49 , 50 . Drought events include meteorological, soil moisture and hydrological (streamflow, groundwater) droughts 51 . The rationale for analysing floods and droughts together is based on their position at the two extremes of the same hydrological cycle, the similarity of some management strategies (for example, warning systems, water reservoir infrastructure), potential trade-offs in the operation of the same infrastructure 52 and more general interactions between these two risks (for example, water supply to illegal settlements that may spur development and therefore flood risk). There may therefore be value in management communities learning from each other 18 .
The dataset comprises: (1) detailed review-style reports about the events and key processes between the events, such as changes in risk management (open access data; Data Availability statement); (2) a key data table that contains the data (qualitative and quantitative) characterizing the indicators for the paired events, extracted from individual reports (open access data); and (3) an overview table providing indicators-of-change between the first and second events (Supplementary Table 3 ). To minimize the elements of subjectivity and uncertainty in the analysis, we (1) used indicators-of-change as opposed to indicators of absolute values, (2) calculated indicators from a set of sub-indicators (Supplementary Table 1 ) and (3) implemented a quality assurance protocol. Commonly, more than one variable was assessed per sub-indicator (for example, flood discharges at more than one stream gauge, or extreme rainfall at several meteorological stations). A combination or selection of the variables was used based on hydrological reasoning on the most relevant piece of information. Special attention was paid to this step during the quality assurance process, drawing on the in-depth expertise on events of one or more of our co-authors. The assignment of values for the indicators-of-change, including quality assurance, was inspired by the Delphi Method 53 that is built on structured discussion and consensus building among experts. The process was driven by a core group (H.K., A.F.V.L., K. Schröter, P.J.W. and G.D.B.) and was undertaken in the following steps: (1) on the basis of the detailed report, a core group member suggested values for all indicators-of-change for a paired event; (2) a second member of the core group reviewed these suggestions; in case of doubt, both core group members rechecked the paired event report and provided a joint suggestion; (3) all suggestions for the indicators-of-change for all paired events were discussed in the core group to improve consistency across paired events; (4) the suggested values of the indicators-of-change were reviewed by the authors of the paired-event report; and finally (5), the complete table of indicators-of-change (Supplementary Table 3 ) was reviewed by all authors to ensure consistency between paired events. Compound events were given special consideration, and the best possible attempt was made to isolate the direct effects of floods and droughts from those of concurrent phenomena on hazard, exposure and impact, based on expert knowledge of the events of one or more of the co-authors. For instance, in the course of this iterative process it became clear that fatalities during drought events were not caused by a lack of water, but by the concurrent heatwave. It was thus decided to omit the sub-indicator ‘fatalities’ in drought impact characterization. The potential biases introduced by compound events were further reduced by the use of the relative indicators-of-change between similar event types with similar importance of concurrent phenomena.
The indicator-of-change of impact is composed of the following sub-indicators: number of fatalities (for floods only), direct economic impact, indirect impact and intangible impact (Supplementary Table 1 ). Flood hazard is composed of the sub-indicators precipitation/weather severity, severity of flood, antecedent conditions (for pluvial and riverine floods only), as well as the following for coastal floods only: tidal level and storm surge. Drought hazard is composed of the duration and severity of drought. Exposure is composed of the two sub-indicators people/area/assets exposed and exposure hotspots. Vulnerability is composed of the four sub-indicators lack of awareness and precaution, lack of preparedness, imperfect official emergency/crisis management and imperfect coping capacity. Indicators-of-change, including sub-indicators, were designed such that consistently positive correlations with impact changes are expected (Supplementary Table 1 ). For instance, a decrease in 'lack of awareness' leads to a decrease in vulnerability and is thus expected to be positively correlated with a decrease in impacts. Management shortcomings are characterized by problems with water management infrastructure and non-structural risk management shortcomings, which means that non-structural measures were not optimally implemented. These sub-indicators were aggregated into indicators-of-change for impact, hazard, exposure, vulnerability and management shortcomings, to enable a consistent comparison between flood and drought paired events. This set of indicators is intended to be as complementary as possible, but overlaps are hard to avoid because of interactions between physical and socioeconomic processes that control flood and drought risk. Although the management shortcoming indicator is primarily related to the planned functioning of risk management measures, and hazard, exposure and vulnerability primarily reflect the concrete effects of measures during specific events, there is some overlap between the management shortcoming indicator and all three drivers. Supplementary Table 1 provides definitions and examples of description or measurement of sub-indicators for flood and drought paired events.
The changes are indicated by −2/2 for large decrease or increase, −1/1 for small decrease or increase and 0 for no change. In the case of quantitative comparisons (for example, precipitation intensities and monetary damage), a change of less than around 50% is usually treated as a small change and above approximately 50% as a large change, but always considering the specific measure and paired events. Supplementary Table 2 provides representative examples from flood and drought paired events showing how differences in quantitative variables and qualitative information between the two events of a pair correspond to the values of the sub-indicators, ranging from large decrease (−2) to large increase (+2). We assume that an event is unprecedented in a subjective way—that is, it has probably not been experienced before—if the second event of a pair is much more hazardous than the first (hazard indicator-of-change +2).
Spearman’s rank correlation coefficients are calculated for impact, drivers and management shortcomings, separated for flood and drought paired events. Despite the measures taken to minimize the subjectivity and uncertainty of indicator assignment, there will always be an element of subjectivity. To address this, we carried out a Monte Carlo analysis (1,000 iterations) to test the sensitivity of the results when randomly selecting 80% of flood and drought paired events. For each subsample correlation, coefficients and P values were calculated to obtain a total of 1,000 correlation and 1,000 P value matrices. The 25th and 75th quantiles of the correlation coefficients and P values were calculated separately (Extended Data Fig. 3 ).
Data availability
The dataset containing the individual paired event reports, the key data table and Supplementary Tables 1 – 3 are openly available via GFZ Data Services ( https://doi.org/10.5880/GFZ.4.4.2022.002 ). Source data are provided with this paper.
Jongman, B. et al. Declining vulnerability to river floods and the global benefits of adaptation. Proc. Natl Acad. Sci. USA 112 , E2271–E2280 (2015).
Article CAS PubMed PubMed Central Google Scholar
Formetta, G. & Feyen, L. Empirical evidence of declining global vulnerability to climate-related hazards. Glob. Environ. Change 57 , 101920 (2019).
Article PubMed PubMed Central Google Scholar
IPCC. Managing the Risks of Extreme Events and Disasters to Advance Climate Change Adaptation (eds Field, C.B. et al.) (Cambridge Univ. Press, 2012).
Bouwer, L. M. Have disaster losses increased due to anthropogenic climate change? Bull. Am. Meteorol. Soc. 92 , 39–46 (2011).
Article ADS Google Scholar
Ward, P. J. et al. Natural hazard risk assessments at the global scale. Nat. Hazards Earth Syst. Sci. 20 , 1069–1096 (2020).
Economic Losses, Poverty & Disasters (1998–2017) (UNISDR (United Nations Office for Disaster Risk Reduction) and CRED (Centre for Research on the Epidemiology of Disasters), 2018); https://www.cred.be/unisdr-and-cred-report-economic-losses-poverty-disasters-1998-2017
Dottori, F. et al. Increased human and economic losses from river flooding with anthropogenic warming. Nat. Clim. Change 8 , 781–786 (2018).
Cammalleri, C. et al. Global Warming and Drought Impacts in the EU (Publications Office of the European Union, 2020); https://doi.org/10.2760/597045
Terminology on Disaster Risk Reduction (UNDRR (United Nations Office for Disaster Risk Reduction), 2017); www.undrr.org/terminology
Cutter, S. L., Boruff, B. J. & Shirley, W. L. Social vulnerability to environmental hazards. Soc. Sci. Q. 84 , 242–261 (2003).
Article Google Scholar
Turner, B. L. et al. A framework for vulnerability analysis in sustainability science. Proc. Natl Acad. Sci. USA 100 , 8074–8079 (2003).
Article ADS CAS PubMed PubMed Central Google Scholar
Eakin, H. & Luers, A. L. Assessing the vulnerability of social-environmental systems. Annu. Rev. Environ. Resour. 31 , 365–394 (2006).
Eriksen, S. et al. Adaptation interventions and their effect on vulnerability in developing countries: help, hindrance or irrelevance? World Dev. Rev. 141 , 105383 (2020).
Kreibich, H. et al. Costing natural hazards. Nat. Clim. Change 4 , 303–306 (2014).
De Ruiter, M. C. et al. Why we can no longer ignore consecutive disasters. Earths Future 8 , e2019EF001425 (2020).
Di Baldassarre, G. A. et al. Perspectives on socio-hydrology: capturing feedbacks between physical and social processes. Water Resour. Res. 51 , 4770–4781 (2015).
Mechler, R. & Bouwer, L. M. Understanding trends and projections of disaster losses and climate change: is vulnerability the missing link? Clim. Change 133 , 23–35 (2015).
Ward, P. J. et al. The need to integrate flood and drought disaster risk reduction strategies. Water Secur. 11 , 100070 (2020).
Raikes, J. et al. Pre-disaster planning and preparedness for floods and droughts: a systematic review. Int. J. Disaster Risk Reduct. 38 , 101207 (2019).
Johnson, K. A. et al. A benefit–cost analysis of floodplain land acquisition for US flood damage reduction. Nat. Sustain. 3 , 56–62 (2019).
Kreibich, H. et al. Adaptation to flood risk: results of international paired flood event studies. Earths Future 5 , 953–965 (2017).
Birkland, T. A. Focusing events, mobilization, and agenda setting. J. Public Policy 18 , 53–74 (1998).
Budiyono, Y. et al. River flood risk in Jakarta under scenarios of future change. Nat. Hazards Earth Syst. Sci. 16 , 757–774 (2016).
Ionita, M. et al. The European 2015 drought from a climatological perspective. Hydrol. Earth Syst. Sci. 21 , 1397–1419 (2017).
Drought Management Plan Report (European Commission, 2007); https://ec.europa.eu/environment/water/quantity/pdf/dmp_report.pdf
Van Lanen, H. A. J. et al. Hydrology needed to manage droughts: the 2015 European case. Hydrol. Process. 30 , 3097–3104 (2016).
Petrucci, O. et al. Civil protection and damaging hydrogeological events: comparative analysis of the 2000 and 2015 events in Calabria (southern Italy). Adv. Geosci. 44 , 101–113 (2017).
NDMC (National Drought Mitigation Center) U.S. Drought Monitor https://droughtmonitor.unl.edu (2020).
Garrick, D. E. et al. Managing the cascading risks of droughts: institutional adaptation in transboundary river basins. Earths Future 6 , 809–827 (2018).
French, A. & Mechler, R. Managing El Niño Risks Under Uncertainty in Peru (International Institute for Applied Systems Analysis, 2017); http://pure.iiasa.ac.at/id/eprint/14849/1/French_Mechler_2017_El%20Ni%C3%B1o_Risk_Peru_Report.pdf
Sörensen, J. & Mobini, S. Pluvial, urban flood mechanisms and characteristics—assessment based on insurance claims. J. Hydrol. 555 , 51–67 (2017).
Muller, M. Cape Town’s drought: don’t blame climate change. Nature 559 , 174–176 (2018).
Article ADS CAS PubMed Google Scholar
White, G. F. Human Adjustment to Floods (Univ. of Chicago Press, 1945).
Wenger, C. Better use and management of levees: reducing flood risk in a changing climate. Environ. Rev. 23 , 240–255 (2015).
Kallis, G. Coevolution in water resource development: the vicious cycle of water supply and demand in Athens, Greece. Ecol. Econ. 69 , 796–809 (2010).
Di Baldassarre, G. et al. Water shortages worsened by reservoir effects. Nat. Sustain. 1 , 617–622 (2018).
Savelli, E. et al. Don’t blame the rain: social power and the 2015–2017 drought in Cape Town. J. Hydrol. 594 , 125953 (2021).
Merz, B. et al. Charting unknown waters—on the role of surprise in flood risk assessment and management. Water Resour.Res. 51 , 6399–6416 (2015).
Brown, A. E. et al. A review of paired catchment studies for determining changes in water yield resulting from alterations in vegetation. J. Hydrol. 310 , 28–61 (2005).
Cutter, S. L. & Finch, C. Temporal and spatial changes in social vulnerability to natural hazards. Proc. Natl Acad. Sci. USA 105 , 2301–2306 (2008).
Hinkel, J. ‘‘Indicators of vulnerability and adaptive capacity’’: towards a clarification of the science–policy interface. Glob. Environ. Change 21 , 198–208 (2011).
Tate, E. Social vulnerability indices: a comparative assessment using uncertainty and sensitivity analysis. Nat. Hazards 63 , 325–347 (2012).
Weichselgartner, J. Disaster mitigation: the concept of vulnerability revisited. Disaster Prev. Manage. 10 , 85–94 (2001).
Adger, W. N. Vulnerability. Glob. Environ. Change 16 , 268–281 (2006).
Birkmann, J. Framing vulnerability, risk and societal responses: the MOVE framework. Nat. Hazards 67 , 193–211 (2013).
Thywissen, K. Components of Risk—a Comparative Glossary (UNU-EHS, 2006); http://collections.unu.edu/view/UNU:1869
Tarasova, L. et al. Causative classification of river flood events. Wiley Interdiscip. Rev. Water 6 , e1353 (2019).
Google Scholar
Rosenzweig, B. R. et al. Pluvial flood risk and opportunities for resilience. Wiley Interdiscip. Rev. Water 5 , e1302 (2018).
Ascott, M. J. et al. Improved understanding of spatio‐temporal controls on regional scale groundwater flooding using hydrograph analysis and impulse response functions. Hydrol. Proc. 31 , 4586–4599 (2017).
Danard, M., Munro, A. & Murty, T. Storm surge hazard in Canada. Nat. Hazards 28 , 407–431 (2003).
Tallaksen, L. & Lanen, H. A. J. V. Hydrological Drought. Processes and Estimation Methods for Streamflow and Groundwater (Elsevier, 2004).
Van den Honert, R. C. & McAneney, J. The 2011 Brisbane floods: causes, impacts and implications. Water 3 , 1149–1173 (2011).
Okoli, C. & Pawlowski, S. D. The Delphi method as a research tool: an example, design considerations and applications. Inform. Manage. 42 , 15–29 (2004).
Download references
Acknowledgements
The presented work was developed by the Panta Rhei Working Groups 'Changes in flood risk' and 'Drought in the Anthropocene' within the framework of the Panta Rhei Research Initiative of the International Association of Hydrological Sciences. We thank the Barcelona Cicle de l’Aigua S.A., Barcelona City Council, Environment Agency (United Kingdom), Länsförsäkringar Skåne, Steering Centre for Urban Flood Control Programme in HCMC (Vietnam), VA SYD and the West Berkshire Council (United Kingdom) for data. The work was partly undertaken under the framework of the following projects: Alexander von Humboldt Foundation Professorship endowed by the German Federal Ministry of Education and Research (BMBF); British Geological Survey’s Groundwater Resources Topic (core science funding); C3-RiskMed (no. PID2020-113638RB-C22), financed by the Ministry of Science and Innovation of Spain; Centre for Climate and Resilience Research (no. ANID/FONDAP/15110009); CNES, through the TOSCA GRANT SWHYM; DECIDER (BMBF, no. 01LZ1703G); Deltares research programme on water resources; Dutch Research Council VIDI grant (no. 016.161.324); FLOOD (no. BMBF 01LP1903E), as part of the ClimXtreme Research Network. Funding was provided by the Dutch Ministry of Economic Affairs and Climate; Global Water Futures programme of University of Saskatchewan; GlobalHydroPressure (Water JPI); HUMID project (no. CGL2017-85687-R, AEI/FEDER, UE); HydroSocialExtremes (ERC Consolidator Grant no. 771678); MYRIAD-EU (European Union’s Horizon 2020 research and innovation programme under grant agreement no. 101003276); PerfectSTORM (no. ERC-2020-StG 948601); Project EFA210/16 PIRAGUA, co-founded by ERDF through the POCTEFA 2014–2020 programme of the European Union; Research project nos. ANID/FSEQ210001 and ANID/NSFC190018, funded by the National Research and Development Agency of Chile; SECurITY (Marie Skłodowska-Curie grant agreement no. 787419); SPATE (FWF project I 4776-N, DFG research group FOR 2416); the UK Natural Environment Research Council-funded project Land Management in Lowland Catchments for Integrated Flood Risk Reduction (LANDWISE, grant no. NE/R004668/1); UK NERC grant no. NE/S013210/1 (RAHU) (W.B.); Vietnam National Foundation for Science and Technology Development under grant no. 105.06-2019.20.; and Vietnam National University–HCMC under grant no. C2018-48-01. D.M. and A. McKenzie publish with the permission of the Director, British Geological Survey. The views expressed in this paper are those of the authors and not the organizations for which they work.
Open access funding provided by Helmholtz-Zentrum Potsdam Deutsches GeoForschungsZentrum - GFZ.
Author information
Authors and affiliations.
GFZ German Research Centre for Geosciences, Section Hydrology, Potsdam, Germany
Heidi Kreibich, Kai Schröter, Nivedita Sairam, Max Steinhausen & Sergiy Vorogushyn
Institute for Environmental Studies, Vrije Universiteit Amsterdam, Amsterdam, the Netherlands
Anne F. Van Loon, Philip J. Ward, Maurizio Mazzoleni, Marlies H. Barendrecht, Anaïs Couasnon & Marleen C. de Ruiter
Leichtweiss Institute for Hydraulic Engineering and Water Resources, Division of Hydrology and River basin management, Technische Universität Braunschweig, Braunschweig, Germany
Kai Schröter
Department of Civil and Environmental Engineering, University of Houston, Houston, TX, USA
Guta Wakbulcho Abeshu & Hong-Yi Li
Lomonosov Moscow State University, Moscow, Russia
Svetlana Agafonova, Natalia Frolova, Maxim Kharlamov, Maria Kireeva & Alexey Sazonov
University of California, Irvine, CA, USA
Amir AghaKouchak & Laurie S. Huning
Department of Civil Engineering, Istanbul Technical University, Istanbul, Turkey
Hafzullah Aksoy
Center for Climate and Resilience Research, Santiago, Chile
Camila Alvarez-Garreton & Mauricio Zambrano-Bigiarini
Department of Civil Engineering, Universidad de La Frontera, Temuco, Chile
Operations Department, Barcelona Cicle de l’Aigua S.A, Barcelona, Spain
Blanca Aznar & Jordi Oriol Grima
Global Institute for Water Security, University of Saskatchewan, Saskatoon, Saskatchewan, Canada
Laila Balkhi, Hayley Carlson & Saman Razavi
LEGOS, Université de Toulouse, CNES, CNRS, IRD, UPS, Toulouse, France
Sylvain Biancamaria
Department of Groundwater Management, Deltares, Delft, the Netherlands
Liduin Bos-Burgering
School of Geography, Earth and Environmental Sciences, University of Birmingham, Birmingham, UK
Chris Bradley & Lucinda Capewell
Agency for the Assessment and Application of Technology, Jakarta, Indonesia
Yus Budiyono
Department of Civil and Environmental Engineering, Imperial College London, London, UK
Wouter Buytaert
Department of Civil Engineering, Beykent University, Istanbul, Turkey
Yonca Cavus
Graduate School, Istanbul Technical University, Istanbul, Turkey
Faculty of Environment and Natural Resources, University of Freiburg, Freiburg, Germany
Yonca Cavus, Mathilde Erfurt, Kerstin Stahl & Michael Stoelzle
Geographical Sciences, University of Bristol, Bristol, UK
Gemma Coxon & Jim Freer
Cabot Institute, University of Bristol, Bristol, UK
Gemma Coxon, Jim Freer & Thorsten Wagener
Department of Agriculture, Hellenic Mediterranean University, Iraklio, Greece
Ioannis Daliakopoulos
Université de Lorraine, LOTERR, Metz, France
Claire Delus & Didier François
CNR-IRPI, Research Institute for Geo-Hydrological Protection, Cosenza, Italy
Giuseppe Esposito & Olga Petrucci
INRAE, Bordeaux Sciences Agro, UMR ISPA, Villenave dʼOrnon, France
Frédéric Frappart
University of Saskatchewan, Centre for Hydrology, Canmore, Alberta, Canada
Environmental Policy and Planning Group, Department of Urban Studies and Planning, Massachusetts Institute of Technology, Cambridge, MA, USA
Animesh K. Gain
Department of Economics, Ca’ Foscari University of Venice, Venice, Italy
Lab of Geophysical-Remote Sensing & Archaeo-environment, Institute for Mediterranean Studies, Foundation for Research and Technology Hellas, Rethymno, Greece
Manolis Grillakis
Pontificia Bolivariana University, Faculty of Civil Engineering, Bucaramanga, Colombia
Diego A. Guzmán
California State University, Long Beach, CA, USA
Laurie S. Huning
Alfred Wegener Institute Helmholtz Center for Polar and Marine Research, Palaeoclimate Dynamics Group, Bremerhaven, Germany
Monica Ionita & Viorica Nagavciuc
Emil Racovita Institute of Speleology, Romanian Academy, Cluj-Napoca, Romania
Monica Ionita
Forest Biometrics Laboratory, Faculty of Forestry, Ștefan cel Mare University, Suceava, Romania
Water Problem Institute Russian Academy of Science, Moscow, Russia
Maxim Kharlamov & Alexey Sazonov
Faculty of Environment, University of Science, Ho Chi Minh City, Vietnam
Dao Nguyen Khoi & Pham Thi Thao Nhi
Environment Agency, Bristol, UK
Natalie Kieboom & Hannah Mathew-Richards
School of Chemical and Environmental Engineering, Technical University of Crete, Chania, Greece
Aristeidis Koutroulis
Servicio Nacional de Meteorología e Hidrología del Perú, Lima, Peru
Waldo Lavado-Casimiro
Department of Applied Physics, University of Barcelona, Barcelona, Spain
María Carmen LLasat
Water Research Institute, University of Barcelona, Barcelona, Spain
British Geological Survey, Wallingford, UK
David Macdonald & Andrew McKenzie
Centre of Natural Hazards and Disaster Science, Uppsala, Sweden
Johanna Mård, Elisa Savelli & Giuliano Di Baldassarre
Department of Earth Sciences, Uppsala University, Uppsala, Sweden
Civil and Environmental Engineering, The Pennsylvania State University, State College, PA, USA
Alfonso Mejia
Escola de Engenharia de Sao Carlos, University of São Paulo, São Paulo, Brasil
Eduardo Mario Mendiondo & Felipe Augusto Arguello Souza
Department of Water Resources & Delta Management, Deltares, Delft, the Netherlands
Marjolein Mens
Trelleborg municipality, Trelleborg, Sweden
Shifteh Mobini
Department of Water Resources Engineering, Lund University, Lund, Sweden
Shifteh Mobini & Johanna Sörensen
University of Potsdam, Institute of Environmental Science and Geography, Potsdam, Germany
Guilherme Samprogna Mohor & Thorsten Wagener
University of Science and Technology of Hanoi, Vietnam Academy of Science and Technology, Hanoi, Vietnam
Thanh Ngo-Duc
Institute for Environment and Resources, Vietnam National University Ho Chi Minh City, Ho Chi Minh City, Vietnam
Thi Thao Nguyen Huynh, Hong Quan Nguyen & Thi Van Thu Tran
Institute for Circular Economy Development, Vietnam National University Ho Chi Minh City, Ho Chi Minh City, Vietnam
Hong Quan Nguyen
Observatori de l’Ebre, Ramon Llull University – CSIC, Roquetes, Spain
Pere Quintana-Seguí
School of Environment and Sustainability, University of Saskatchewan, Saskatoon, Saskatchewan, Canada
Saman Razavi
Department of Civil, Geological and Environmental Engineering, University of Saskatchewan, Saskatoon, Saskatchewan, Canada
Dipartimento di Ingegneria Civile, Edile e Ambientale, Sapienza Università di Roma, Rome, Italy
Elena Ridolfi
University of Applied Sciences, Magdeburg, Germany
Jannik Riegel
Center for Environmental and Geographic Information Services, Dhaka, Bangladesh
Md Shibly Sadik
Earth and Environmental Systems Institute, The Pennsylvania State University, State College, PA, USA
Sanjib Sharma
Institute of Meteorology and Water Management National Research Institute, Warsaw, Poland
Wiwiana Szalińska & Tamara Tokarczyk
Key Laboratory of Water Cycle and Related Land Surface Processes, Institute of Geographical Sciences and Natural Resources Research, Chinese Academy of Sciences, Beijing, China
Qiuhong Tang & Yueling Wang
Department of Hydraulic Engineering, Tsinghua University, Beijing, China
Fuqiang Tian
Royal Botanical Gardens Kew, London, UK
Carolina Tovar
KWR Water Research Institute, Nieuwegein, the Netherlands
Marjolein H. J. Van Huijgevoort
Department of Physical Geography, Utrecht University, Utrecht, the Netherlands
Michelle T. H. van Vliet
Civil Engineering, University of Bristol, Bristol, UK
Thorsten Wagener & Doris E. Wendt
School of Natural Resources, University of Nebraska-Lincoln, Lincoln, NE, USA
Elliot Wickham
School of Geography and Ocean Science, Nanjing University, Nanjing, China
Institute of Hydraulic Engineering and Water Resources Management, Technische Universität Wien, Vienna, Austria
Günter Blöschl
Department of Integrated Water Systems and Governance, IHE Delft, Delft, the Netherlands
Giuliano Di Baldassarre
You can also search for this author in PubMed Google Scholar
Contributions
H.K. initiated the study and led the work. H.K., A.F.V.L., K. Schröter, P.J.W. and G.D.B. coordinated data collection, designed the study and undertook analyses. All co-authors contributed data and provided conclusions and a synthesis of their case study (the authors of each paired event report were responsible for their case study). M. Mazzoleni additionally designed the figures, and he and N.S. contributed to the analyses. H.K., G.D.B., P.J.W., A.F.V.L., K. Schröter and G.D.B. wrote the manuscript with valuable contributions from all co-authors.
Corresponding author
Correspondence to Heidi Kreibich .
Ethics declarations
Competing interests.
The authors declare no competing interests.
Peer review
Peer review information.
Nature thanks Elizabeth Tellman, Oliver Wing and the other, anonymous, reviewer(s) for their contribution to the peer review of this work.
Additional information
Publisher’s note Springer Nature remains neutral with regard to jurisdictional claims in published maps and institutional affiliations.
Extended data figures and tables
Extended data fig. 1 location of flood and drought paired events coloured according to their indicators-of-change..
a , Change in hazard; b , change in exposure; c , change in vulnerability and d , change in management shortcomings.
Extended Data Fig. 2 Parallel plot of paired events with the same hazard of both events.
The hazard change is zero for all shown paired events. The lines show how the different combinations of indicators-of-change result in varying changes in impacts. Small offsets within the grey bars of the indicator-of-change values enable the visualization of all lines.
Extended Data Fig. 3 Results of the sensitivity analyses.
a–d Correlation matrix of indicators-of-change for 25th and 75th quantiles of correlation coefficients and p-values, respectively ( a , c ) and 75th and 25th quantiles of correlation coefficients and p-values, respectively ( b , d ) separate for flood and drought paired events. Quantiles of correlation coefficients and p-values were calculated separately; colours of squares indicate Spearman’s rank correlation coefficients; sizes of squares indicates p-values. Fig. 2a, c is added to the right to ease comparison.
Extended Data Fig. 4 Theoretical framework used in this study (adapted from IPCC 3 ).
This theoretical risk framework considers impact as a result of three risk components or drivers: hazard, exposure and vulnerability, which in turn are modified by management.
Supplementary information
Supplementary tables.
Supplementary Tables 1–3.
Source Data Fig. 1.
Source data fig. 2., source data fig. 3., source data extended data fig. 1., source data extended data fig. 2., source data extended data fig. 3., rights and permissions.
Open Access This article is licensed under a Creative Commons Attribution 4.0 International License, which permits use, sharing, adaptation, distribution and reproduction in any medium or format, as long as you give appropriate credit to the original author(s) and the source, provide a link to the Creative Commons license, and indicate if changes were made. The images or other third party material in this article are included in the article’s Creative Commons license, unless indicated otherwise in a credit line to the material. If material is not included in the article’s Creative Commons license and your intended use is not permitted by statutory regulation or exceeds the permitted use, you will need to obtain permission directly from the copyright holder. To view a copy of this license, visit http://creativecommons.org/licenses/by/4.0/ .
Reprints and permissions
About this article
Cite this article.
Kreibich, H., Van Loon, A.F., Schröter, K. et al. The challenge of unprecedented floods and droughts in risk management. Nature 608 , 80–86 (2022). https://doi.org/10.1038/s41586-022-04917-5
Download citation
Received : 19 August 2021
Accepted : 30 May 2022
Published : 03 August 2022
Issue Date : 04 August 2022
DOI : https://doi.org/10.1038/s41586-022-04917-5
Share this article
Anyone you share the following link with will be able to read this content:
Sorry, a shareable link is not currently available for this article.
Provided by the Springer Nature SharedIt content-sharing initiative
This article is cited by
Associations between a cash voucher intervention, food consumption, and coping strategies in somali food-insecure populations.
- Theresa Fang
- Yunhee Kang
Agriculture & Food Security (2024)
Limited progress in global reduction of vulnerability to flood impacts over the past two decades
- Inga J. Sauer
- Benedikt Mester
- Christian Otto
Communications Earth & Environment (2024)
Application of a hybrid algorithm of LSTM and Transformer based on random search optimization for improving rainfall-runoff simulation
- Wenzhong Li
- Chengshuai Liu
Scientific Reports (2024)
Strategic storm flood evacuation planning for large coastal cities enables more effective transfer of elderly populations
- Mingfu Guan
Nature Water (2024)
Applying a machine learning-based method for the prediction of suspended sediment concentration in the Red river basin
- Son Q. Nguyen
- Linh C. Nguyen
- Sylvain Ouillon
Modeling Earth Systems and Environment (2024)
By submitting a comment you agree to abide by our Terms and Community Guidelines . If you find something abusive or that does not comply with our terms or guidelines please flag it as inappropriate.
Quick links
- Explore articles by subject
- Guide to authors
- Editorial policies
Sign up for the Nature Briefing newsletter — what matters in science, free to your inbox daily.


An official website of the United States government
The .gov means it’s official. Federal government websites often end in .gov or .mil. Before sharing sensitive information, make sure you’re on a federal government site.
The site is secure. The https:// ensures that you are connecting to the official website and that any information you provide is encrypted and transmitted securely.
- Publications
- Account settings
Preview improvements coming to the PMC website in October 2024. Learn More or Try it out now .
- Advanced Search
- Journal List
Effective adaptation to rising flood risk
Brenden jongman.
1 Institute for Environmental Studies, VU University Amsterdam, Amsterdam, The Netherlands
2 Global Facility for Disaster Reduction and Recovery (GFDRR), The World Bank, Washington, DC USA
Floods are causing increasing havoc in our rapidly urbanizing world, with disproportionally high impacts on the poorest and most vulnerable. Effective adaptation strategies are needed, which combine flood protection infrastructure, nature-based solutions, and risk financing schemes to manage floods and buffer their economic impacts.
Global weather-related disaster losses exceeded US$300 billion in 2017, which made this the most costly year on record and continues a long-term upward trend 1 . For the first time in history, over half the world’s population now live in cities, many of which are located at rivers, along coastlines, or both. A new study shows that the total urban area exposed to flooding in Europe has increased by 1000% over the past 150 years 2 . On a global scale, trends in flood zone urbanization have been similarly steep and continue to climb, especially in Africa and Asia 3 , 4 . Not only does this mean that ever more human assets are in the way of floods, but urbanization with an increase of non-permeable surfaces and lack of natural drainage creates additional flooding issues that did not previously exist.
Socioeconomic changes are further compounded by climate change-induced increases in extreme rainfall which amplify the intensity and probability of floods. In Europe, all climate models consistently show an increase in flood impacts across most Western and Central countries, approximately doubling the expected damage by the end of the century 5 . In addition, changing patterns of spring snowmelt and winter storms have dramatically affected the timing of floods throughout the year 6 . Within these long-term trends, inter-annual fluctuations due to large scale atmospheric patterns such as the North Atlantic Oscillation (NAO) and the East Atlantic pattern (EA) cause strong year-to-year fluctuations in rainfall and resulting flood damages 7 .
The impacts of flooding go far beyond direct damages to assets and infrastructure. Economic losses resulting from business disruption, welfare effects and supply chain shocks can often at times equal or exceed direct damages 8 . In extreme cases, such as the shut-down of Wall Street due to Hurricane Sandy, economic ripple effects may be felt across an entire sector around the globe. The welfare loss from flood events hits the poorest in society hardest. In many countries, the poorest population groups are relatively overexposed to flooding, as they are often forced to live and work in low-lying areas 9 . In addition, the poorest households are more vulnerable to the resulting impacts to their income, and can often be pushed across the poverty line by a single event 10 . As such, natural disasters may effectively increase global poverty 11 .
Positive trends, however, are visible. Economic development, technological progress and targeted adaptation interventions help reduce flood impacts over time. In Europe, fatalities and normalized economic losses (losses as % of GDP) have decreased significantly over recent decades, despite an increase in flooded area and absolute losses 2 . Globally, too, such a decline in relative impact has been observed, as low-income countries are becoming less vulnerable as per-capita income rises 12 . This demonstrates that effective adaptation to flooding is feasible, even when faced by growing exposure and a changing climate.
Adaptation to flooding
If all natural disasters could suddenly and completely be eliminated, hundreds of billions of dollars in damages would be saved each year and the number of people living in extreme poverty would immediately fall by 26 million 11 . However, not all disasters can be prevented. Effective adaptation to rising flood risk requires a diversified approach of interventions, which may include structural flood protection measures, early warning systems, risk-informed land planning, nature-based solutions, social protection and risk financing instruments 13 . The right mix of measures varies from place to place, subject to levels of risk, funding, and political will.
Physical flood protection measures, such as dikes and levees, are generally cost-effective in areas with high population and asset concentrations 14 . The Netherlands, being a highly populated and highly flood prone country, is the prime example of a country relying heavily on such structural measures. Most of the coastline is protected with a dike system offering protection against events that only occur once every 10,000 years. However, such protection works require immense capital investments for construction and maintenance, for which both political momentum and government budgets are often missing. In addition, research in the field of socio-hydrology has shown that increasing flood protection can give a false sense of security and may boost development in these protected flood-prone areas. However, while the resulting system may have a lower risk overall, the potential impacts of a dike-breaching event can be catastrophic 15 .
Recently, governments are increasingly turning to nature to manage flooding 16 . Such nature-based solutions include widening of natural flood plains, protecting and expanding wetlands, restoring oyster and coral reefs and investing in urban green spaces to reduce run-off. In the United States, natural wetlands have moderated damages from Hurricane Sandy by an estimated $625 million 17 . In the Gulf of Mexico, nature-based adaptation measures could even reduce overall risk by a stunning $50 billion, with an average benefit to cost ratio of 3.5 18 . Meanwhile, China has started implementing the national “Sponge Cities” program in 16 pilot cities, where vast amounts of green space will be integrated into urban design to prevent surface flooding. In addition to effectively reducing flood risk, nature-based solutions can have a wide range of positive effects on ecosystem conservation, carbon storage, tourism and local employment. Implementing natural approaches often also requires the involvement of various stakeholder groups, thereby helping with awareness raising and consensus building.
Yet, in spite of the multiple benefits of nature-based solutions, flood control remains heavily dependent on ‘gray’ infrastructure interventions. The relatively slow uptake of nature-based solutions demonstrates the current lack of understanding regarding feasible protection levels, appropriate maintenance and monitoring schemes, and available flexible funding mechanisms for such approaches 19 . In many cases, combining green and gray infrastructure measures into so-called ‘hybrid’ solutions have the best potential to safeguard the security provided by infrastructure while providing the benefits of natural approaches (Fig. 1 ). Careful analyses should be conducted to evaluate the range of available options and design flood management schemes that combine natural, infrastructural and policy instruments in the most effective way 20 .

Hybrid flooding solutions. By reducing wave run-up, a hybrid solution that combines mangrove conservation with a levee can reduce construction and maintenance costs while offering the same protection level. Figure reproduced from ref. 20
Financing the residual risk
Whereas adaptation has ensured that normalized losses as a percentage of GDP are stable, or even declining in some regions, the absolute financial loss levels are still on the rise. Financing the repair of and recovery from flood damages has therefore become a rising challenge. Uninsured losses and the lack of financial means for flood recovery and response may impact peoples’ well-being, the country’s budget and the overall economy. The construction of risk financing strategies determines the short-term and long-term financial burden of floods on individuals and companies. If effectively designed, risk transfer mechanisms such as insurance products can even incentivize active risk reduction by policy holders.
Individual European countries have put in place a variety of national risk financing systems, including differing private flood insurance products. However, the insurance coverage rates are generally low, and cannot work independently without a parallel government mechanism. Government support can include subsidizing insurance premiums, managing insurance schemes, acting as a monopolistic insurance provider, or simply providing ad-hoc post-disaster aid 21 . The latter is often associated with negative effects, such as reduced incentives for risk reduction.
On a continental level, the European Commission also provides financial relief through the EU Solidarity Fund. This Fund can supplement the financial expenditures of member states for large events. However, the Solidarity Fund is insufficient to cover all eligible losses and does not encourage risk reduction 22 . Under climate change induced increases in flood extremes, the financial sustainability of the Fund is likely to deteriorate further. Europe, however, is not the only place where financing schemes are dependent on accurate understanding of risk trends. In the United States, new modeling work has shown that the official national flood maps may underestimate flood exposure by a factor 3, which could have important consequences for the national insurance program 23 .
Risk perception
The design of such holistic risk management strategies requires an accurate understanding of the level of risk across the various layers of society. One important remaining limitation in our understanding of flood risk is the way individuals perceive and respond to risk. Even if we manage to model population density and flood inundation with increasing accuracy, assumptions about peoples’ risk reducing behavior, willingness to relocate, and access to information play a key role in the actual level of risk. Neglecting this behavioral component may overestimate actual impacts by a factor two 25 . Recent innovations in agent-based modeling now allow us to integrate complex human behavior in integrated risk assessments, which will allow us to create much more realistic scenarios of flood impacts and possible adaptation solutions 26 .
The new age of social media may help us better understand the human aspects of flooding. All Twitter messages are now automatically screened and georeferenced in order to identify and locate flood events around the world 24 (Fig. 2 ). The Philippines Red Cross is already using this as an operational tool for flood response, opening the door not only to the earlier detection of events, but also to understanding the perception, impacts and response to floods as they occur. In areas where Twitter is not available, similar rapid text-mining technologies are currently being developed that rely on websites, newspaper articles, and text messages.

Social media and flooding. Number of flood-related Twitter messages (“tweets”) during the January 2018 floods in France. Advances in social media analytics are used to detect and monitor floods earlier, allowing for more effective response (figure produced by the author using data from the TAGGS 24 model, accessible through the Global Flood Monitor - https://www.globalfloodmonitor.org/ )
Clearly, managing flood risk involves much more than building dikes. Governments need to be invested in the complex task of adopting holistic risk management strategies that combine economically viable investments in risk reduction along with well-designed financial instruments to cover residual losses, whilst acknowledging the ever-changing and cross-boundary nature of risk.
Acknowledgements
I thank J de Bruijn and B van Wesenbeeck for helpful discussions and input.
Author contributions
B.J. wrote the manuscript.
Competing interests
The author declares no competing interests.
Publisher's note: Springer Nature remains neutral with regard to jurisdictional claims in published maps and institutional affiliations.
- Arts & Culture
Get Involved
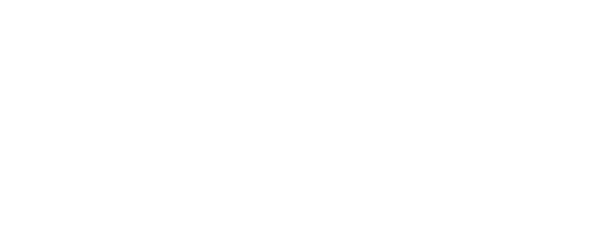
Autumn 2023
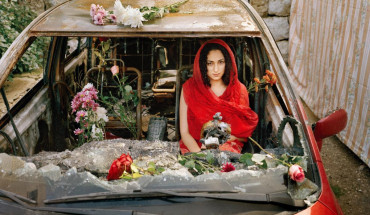
Exhibition Opening: Louder than Hearts, Women Photographers from the Arab World and Iran
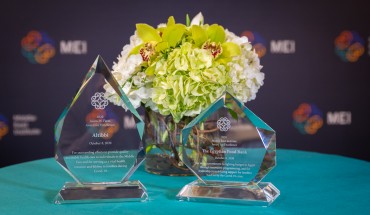
Annual Gala Dinner
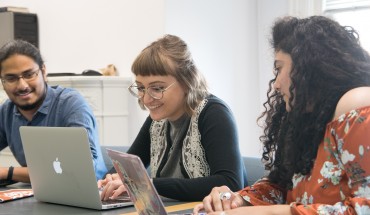
Internships
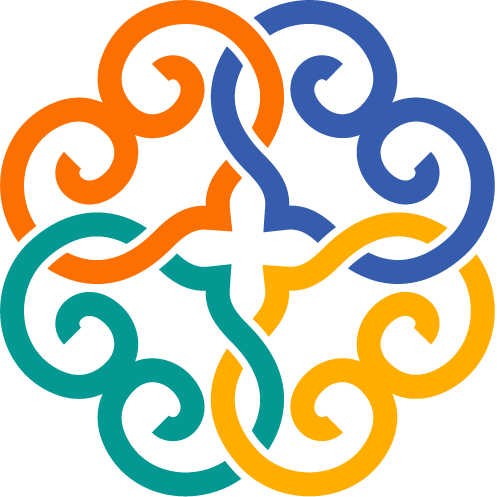
Addressing Worldwide Flood Concerns: Empowering Local Communities
Denise Corsel
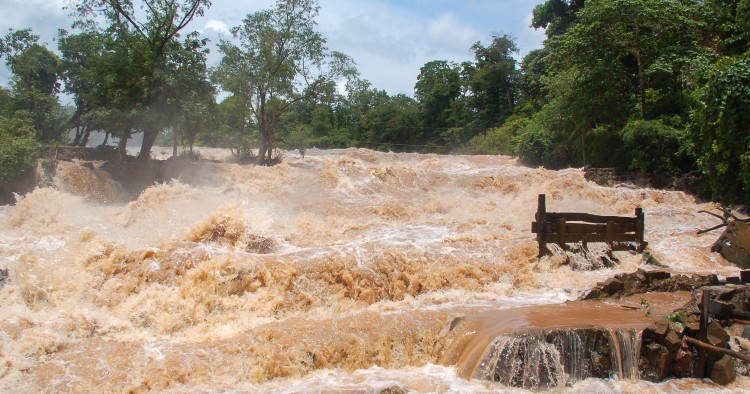
Over the last 25 years, the world has seen a rise in the frequency of natural disasters in rich and poor countries alike. Today, more people than ever are at risk from natural hazards, with those in developing countries particularly at risk. This essay series is intended to explore measures that have been taken, and could be taken, in order to improve responses to the threat or occurrence of natural disasters in the MENA and Indo-Pacific regions. Read more ...
Floods have become a growing concern throughout the world. [1] Fueling this concern is the prediction that climate change will increase the intensity and severity of flooding. [2] There are also growing concerns that climate change will dramatically increase the health risks associated with contaminated water and dangerous substances that are released during floods. [3] These concerns need to be addressed in order to reduce the negative impact of floods upon communities worldwide.
Coping with floods is a major challenge for many communities, especially those in developing nations, which generally have to manage the responses to and recovery from them mostly on their own. Governments, NGOs and other organizations provide aid during a natural disaster when, where and how they can. However, their extent of their contributions sometimes fail to meet the needs of local communities, especially immediately following the disaster but also in the longer term, due to their limited financial capabilities, inadequate access to affected areas, lack of awareness or political constraints under which they operate.
This essay focuses on the role of local communities during the recovery stage from floods. By drawing on a case study of rural communities in Laos, [4] the essay highlights the potential benefits of equipping local communities with long-term practices that will assist them in recovery efforts. The essay concludes with a discussion of the lessons and insights gained from this case and that they could be applied in other countries to improve flood resiliency.
The Importance of a Community-Centered Approach
This essay discusses floods from the perspective of long-term recovery since the latter contributes to resiliency, which is defined as “the ability to recover readily.” [5] The recovery stage—encompassing the process and outcome—is crucial because it covers everything that is required for the affected community to return to conditions of normality. [6] Floods are almost impossible to prevent and the scale of adverse consequences from floods is exceedingly difficult to gauge. Therefore, mitigating the consequences of floods is often quite challenging, especially in rural villages in developing countries, where technology and finances are in short supply. This overall magnifies the importance of the recovery stage.
Local communities are directly affected by floods and thus are the primary risk-bearers in such situations. They are the first on the scene and generally carry out the initial response. It is thus important to focus particularly on local communities during their recovery. Floods can inflict enormous damage, [7] especially upon those who are the most vulnerable community members. The damage affects property, finances, job security, emotional and health status, and livelihoods. Therefore, the community affected has an investment and a strong motive to recover and to return to normal life as quickly as possible. Compared to others, such as aid organizations, supporters and governments, the community itself will prioritize the recovery process the most. Therefore, a community-centered approach to improve flood resiliency is recommended. The Sendai Framework for Disaster Risk Reduction [8] supports this by emphasizing that there should be a people-centered approach when preventing disaster risk. To do so, during the recovery stage of a flood it is important for people to be both reflective and pro-active so that they can improve the management of future flooding.
Another reason why a community-centered approach is recommended is because with the increase of natural disasters throughout the world, [9] aid response will not be enough on its own. Resilient development [10] is, therefore, more important now than ever before. Developing the community to be resilient will not only enhance the efficiency of the recovery, but will assist with building a stronger system that can better manage all types of risks while improving the chances of maintaining the progress made by the community. Overall it will strengthen the current links between aid organizations and the communities’ development work, which allows for a lasting change. [11]
A community-centered approach can be highly efficient since communities know and understand their own situations best. Some would argue that experts possess greater expertise, theoretical knowledge and specific skills than the communities they seek to assist. Nonetheless, the local public has more everyday knowledge regarding their own surroundings and how their community members are likely to respond to certain recovery actions, which may be crucial to recover efficiently. Additionally, when preparing for a potential flood disaster, the community is the only party that has a clear emotional investment regarding the potential impacts and risks. In any event, it is imperative that all relevant stakeholders are included and participate to have a successful recovery process and outcome. [12] Community participation will enable more effective and long-term flood practices, improve the overall flood management process in place and allow for safer and quicker decisions to be made.
Helping to equip local communities, including their most vulnerable members, with recovery practices will enable them in the long-term to augment their own resilience to floods, which will be especially useful in case the prediction [13] that climate change will produce more intense and severe flooding is correct. As illustrated in the case of Laos’ rural communities, local communities, specifically those that are prone to flooding, tend to already have several recovery practices in place that could, and should be bolstered through the contributions of experts, government authorities and aid.
Recovery Practices in Laos
While numerous flood recovery practices are used throughout the world, local communities in Laos have their own practices, especially since they often experience flooding due to the annual rainy season. Flooding is Laos’ main hazard [14] that affects communities all across the county and often develops into a disaster. [15] Therefore, Laos’ coping practices have developed over many generations. They include traditional practices such as constructing houses on stilts; setting up community banks of rice; using traditional medicines; seeking shelter in temples and/or schools; creating dug-out drainage systems for paddy fields; and removing water from houses with baskets. These practices do indeed help with recovery, though they are predominantly needs-based coping strategies. However, NGOs and United Nations staff are teaching and/or sharing with rural communities other, more advanced flood resilience practices, such as using pumps to remove water; creating official village disaster committees and disaster preparedness plans; employing sturdier home building and repair techniques (e.g. using concrete or stronger wood); and implementing food and drinking storage systems.
In Laos, the management of flooding, which is challenging due to unpredictable flash floods, is primarily conducted at local levels and appears to be effective due to enhanced focus on villages’ own disaster management committees. Owing to the country’s financial situation, [16] flood management is mostly conducted by the individual village, which has its own village chief and elder. Often when a flood occurs, it is not possible for outsiders, including government officials, to enter/reach the villages affected. Therefore, it is important to focus particularly on local communities’ own coping mechanisms during recovery. In such a situation, Laos’ citizens currently manage and work together as a community to manage floods. So far, this has been working satisfactorily. However, with the building of houses in floodplains, logging trees and signs that changing climate is increasing the severity of floods and droughts, [17] existing local coping mechanisms might not be enough. Yet, whether their current practices are adequate to meet these immediate challenges or not, equipping Laos’ citizens with additional recovery practices would be highly beneficial, as it would allow the communities to better manage the (current and future) physical, emotional and secondary damage created by flooding disasters by strengthening their resilience. Additionally, it would allow them to handle the floods better in the long-term, especially if flooding worsens.
Laos’ villagers could learn from other recovery practices used in other countries. For instance, in Khammouane Province (Laos) the main concern during the recovery stage is health since floods spread disease and drinking and washing water become contaminated. This issue could be mitigated by chemically treating the water, as is done in Tanzania [18] or by using water purification tablets, as is done in Bangladesh. [19] Furthermore, another recovery practice that the majority of communities throughout Laos would benefit from would be the introduction of a rice variety that can withstand flooding because currently during floods the loss of rice, the main source of their livelihood, [20] is reducing their capability to recover. This practice has been effective in India, Bangladesh and Nepal, [21] and research is being conducted on how this would work in Laos. [22] There are many other practices that may be helpful, such as in Thailand, where communities are involved with hazard mapping to enhance education, [23] and in Vietnam, where they use schools to hold flood safety campaigns. [24] The idea that building on current practices while introducing new ones to enhance communities’ resilience is gathering momentum.
Nevertheless, it is important that communities are supported in selecting the most beneficial and realistic recovery practices. Additionally, it is crucial that the practices are manageable and accessible by the communities over the long term, thereby ensuring that they are sustainable. As a result, the communities would be empowered and capable of recovering from floods better and quicker, as well as potentially recovering from other natural disasters because some of these practices are transferable.
Some of Laos’ citizens may be content with the way floods are currently managed; however, most are open to learning and to trying out new practices and/or enhancing their current recovery practices. Moreover, villages, such as those in Laos, where residents have learned to cope with disasters on their own and thus, a community-centered approach is already in place, are likely to be more open to strengthening and building on their current approach than to acceding to outsiders’ management of the recovery stage. In fact, Laos’ government, in apparent recognition of this, supports village disaster management committees. Several aid organizations, including the Global Facility for Disaster Reduction and Recovery [25] and the United Nations also promote sustainable assistance to ensure that long-term resilience is strengthened in such a manner that the country and its communities will be better able to manage disasters and ‘build back better’ [26] without them.
Equipping local communities with long-term recovery practices, some of which may be learned from other countries and adapted to country-specific circumstances could contribute to more effective disaster recovery, a better flood management process, and safer and quicker decisions. The main lesson learned from the case study of rural communities in Laos is that in countries where aid is not always readily accessible and where a community-centered management approach has been forming over the years, it is important to focus on and enhance the community-centered approach during the flood recovery stage. Given that the incidence of flooding has increased and is predicted to worsen in countries, including Laos, [27] special attention should be devoted to strengthening long-term local recovery practices, which is part of resilient development. In particular, as also illustrated by this case study, it is crucial to consider sustainability when improving flood resilience at local levels.
Undeniably government agencies and aid organizations play a vital role in disaster management. Nevertheless, they should focus on enhancing the communities’ resilient development so that, in the future, communities can manage floods better on their own and conduct the recovery process with greater efficiency. Particularly during the recovery stage, the risk-bearers should be principally in charge of managing their situation, as it is they who know best what is required to return the community to a state of ‘normality’ as quickly as possible. Where possible, the risk-bearers should be supported in their efforts to guide ‘building back better’ initiatives. [28] In sum, attention should be focused more sharply upon, and more support and resources should be invested in local communities to improve their flood resilience by enhancing their current recovery practices and introducing new ones.
[1] Dawei Han, Flood Risk Assessment and Management (Sharjah: Bentham Science Publishers, 2011) 1.
[2] Duncan Geere, “Global Flooding Risk Could Triple by 2030,” Wired , March 5, 2015, accessed April 15, 2016, http://www.wired.co.uk/news/archive/2015-03/05/flooding-predictions-2030 ; and Tianyi Luo , Andrew Maddocks , Charles Iceland , Philip Ward and Hessel Winsemius, “World’s 15 Countries with the Most People Exposed to River Floods,” World Resources Institute , March 5, 2015, accessed April 15, 2016, http://www.wri.org/blog/2015/03/world%E2%80%99s-15-countries-most-peopl… .
[3] Roger Few and Franziska Matthies, eds ., Flood Hazards & Health: Responding to Present and Future Risks (London: Earthscan, 2006); and National Geographic, “Floods,” National Geographic , 2015, accessed June 26, 2015, http://environment.nationalgeographic.com/environment/natural-disasters… .
[4] Laos, located in South-East Asia, is officially known as Lao PDR, which is short for Lao People’s Democratic Republic.
[5] Michael Pitt, Learning Lessons from the 2007 Floods: An Independent Review by Sir Michael Pitt (London: Cabinet Office, 2008) 349, accessed April 15, 2016, http://webarchive.nationalarchives.gov.uk/20100807034701/http:/archive… .
[6] Home Office, Dealing with Disaster (London: Cabinet Office, 1998), accessed April 15, 2016, http://webarchive.nationalarchives.gov.uk/20050523205851/http:/ukresili… .
[7] Roger Few and Franziska Matthies, eds ., Flood Hazards & Health: Responding to Present and Future Risks (London: Earthscan, 2006).
[8] United Nations Office for Disaster Risk Reduction (U.N.I.S.D.R.), Sendai Framework for Disaster Risk Reduction 2015-2030 (2015) 10, accessed April 15, 2016, http://www.unisdr.org/files/43291_sendaiframeworkfordrren.pdf .
[9] International Federation of Red Cross and Red Crescent Societies, Introduction to the Guidelines for the Domestic Facilitation and Regulation of International Disaster Relief and Initial Recovery Assistance (Geneva: International Federation of Red Cross and Red Crescent Societies, 2011) 3.
[10] Natalia Adler, “Resilient Development Means Better Preparing Children, Families and Communities for Shocks, Making Sure They Can Better Withstand Them, and Helping Them to Recover Quickly,” U.N.I.C.E.F. Technical Note: Resilient Development (2016) 3.
[12] Upala Banerjee, “Adopting Rights-Based Programming Strategies Towards Developing Capacities for Accessing Sustainable Water and Sanitation Facilities: The NAM SAAT/Sida/UNICEF Partnership in Luang Prabang Province in Laos - A Case Study” (U.N.D.P., 2005) 249, accessed May 15, 2016, http://www.unescobkk.org/fileadmin/user_upload/appeal/LLP/LLP_Documenta… .
[13] Duncan Geere, “Global Flooding Risk Could Triple by 2030,” Wired , March 5, 2015, accessed April 15, 2016, http://www.wired.co.uk/news/archive/2015-03/05/flooding-predictions-2030 .
[14] United Nations Office for Disaster Risk Reduction (U.N.I.S.D.R.), Country Assessment Report for Lao PDR: Strengthening of Hydrometeorology Services in Southeast Asia (2013), accessed April 15, 2016, http://www.unisdr.org/files/33988_countryassessmentreportlaopdr[1].pdf .
[15] Saysoth Keoduangsine, Robert Goodwin and Paul Gardner-Stephen, “A Study of an SMS-Based Flood Warning System for Flood Risk Areas in Laos,” International Journal of Future Computer and Communication 3 (2014): 182-186, accessed May 15, 2016, http://www.ijfcc.org/papers/292-M052.pdf .
[16] The World Bank, “Lao PDR Overview,” last modified April 2016, http://www.worldbank.org/en/country/lao/overview .
[17] “Laos Yet to Formulate Drought Response Plan,” Vientiane Times , April 29, 2016, accessed May 11, 2016, http://www.vientianetimes.org.la/FreeContent/FreeConten_Laosyet.htm .
[18] Tumpale Sakijege, John Lupala and Shaaban Sheuya, “Flooding, Flood Risks and Coping Strategies in Urban Informal Residential Areas: The Case of Keko Machungwa, Dar es Salaam, Tanzania,” J àmbá: Journal of Disaster Risk Studies 4 (2012): 1-10, accessed April 15, 2016, doi: 10.4102/jamba.v4i1.46.
[19] S. Paul and J. Routray, “Flood Proneness and Coping Strategies: The Experiences of Two Villages in Bangladesh,” Disasters 34 (2010): 489-508, accessed April 15, 2016, doi: 10.1111/j.1467-7717.2009.01139.x.
[20] “Lao PDR,” The International Rice Research Institute (2015), accessed April 15, 2016, http://irri.org/our-work/locations/lao-pdr .
[21] Amy Kazmin, “Asia Races to Find Drought-Resistant Rice,” Future of the Food Industry , last modified January 13, 2016, http://www.ft.com/cms/s/2/dea46c3e-982a-11e5-9228-87e603d47bdc.html .
[22] “Lao PDR,” The International Rice Research Institute (2015), accessed April 15, 2016, http://irri.org/our-work/locations/lao-pdr .
[23] A. Phonmart, “Promoting Community Awareness and Strengthening Community Resilience.” Presentation by Department of Disaster Prevention and Mitigation (D.D.P.M.), (Bangkok: Ministry of Interior).
[24] Standing Office of Tien Giang Provincial Committee for Flood and Storm Control (P.C.F.S.C.), “Activities under MRC – ADPC – ECHO Project and Achievement . ” Presentation at Regional Workshop, Tien Giang, Laos, 2009.
[25] World Bank, Resilient Recovery: An Imperative for Sustainable Development (2015), accessed May 8, 2016, https://www.gfdrr.org/sites/gfdrr/files/publication/Resilient-Recovery-An-Imperative-for-Sustainable-Development.pdf .
[26] United Nations Lao PDR, “UN Disaster Risk Reduction Chief: Building Back Better Makes Communities More Resilient,” October 9, 2012, accessed April 15, 2016, http://www.la.one.un.org/media-center/news-and-features/20-un-disaster-risk-reduction-chief-building-back-better-makes-communities-more-resilient .
[27] “Laos: Floods highlight disaster-preparedness needs,” IRIN , September 7, 2011, accessed May 7, 2016, http://www.irinnews.org/fr/report/93672/laos-floods-highlight-disasterp… .
[28] United Nations Lao PDR, “UN Disaster Risk Reduction Chief: Building Back Better Makes Communities More Resilient.”
The Middle East Institute (MEI) is an independent, non-partisan, non-for-profit, educational organization. It does not engage in advocacy and its scholars’ opinions are their own. MEI welcomes financial donations, but retains sole editorial control over its work and its publications reflect only the authors’ views. For a listing of MEI donors, please click here .
- 0 Shopping Cart
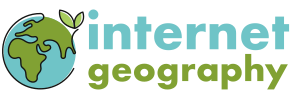
What are the human and physical factors that affect flood risk?
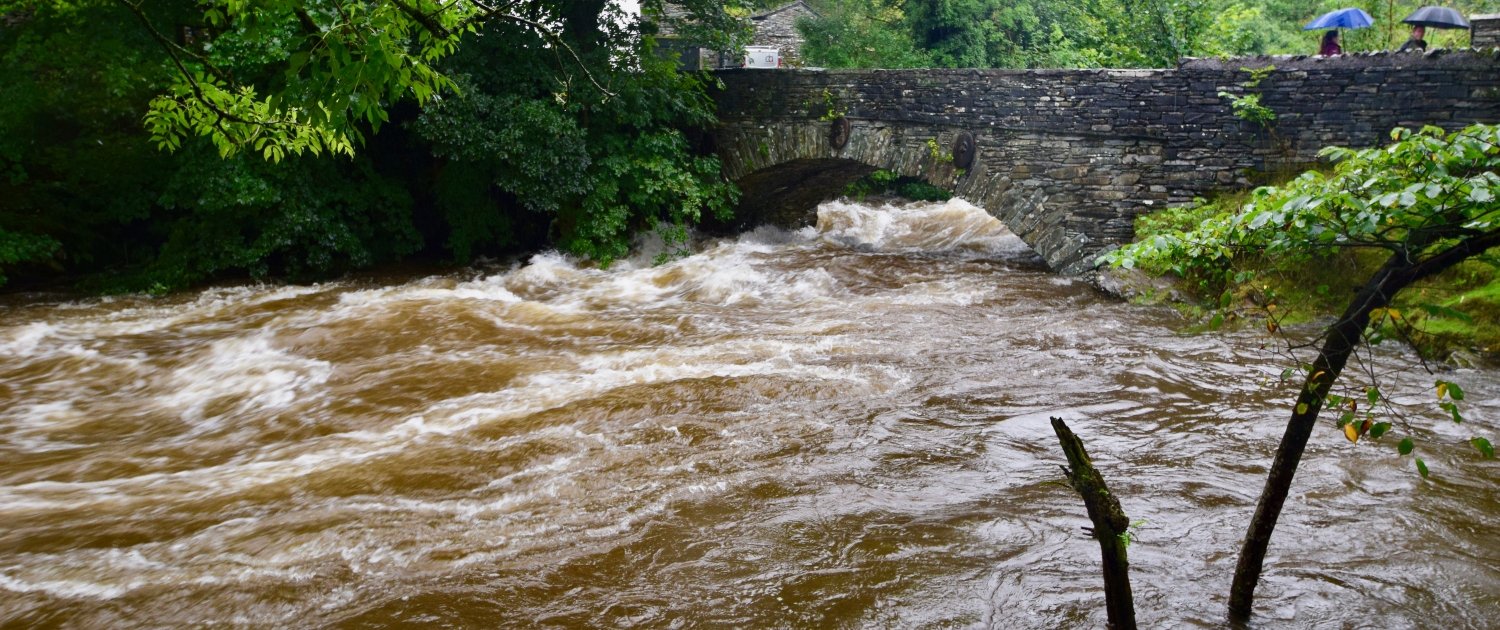
River discharge is the volume of water that flows in a river channel and is measured in cubic metres per second. An increase in discharge causes river levels to increase. Flooding occurs when the bankfull capacity of a river is exceeded (water spills over the banks of the river). There are a range of physical and human factors that affect flood risk.
What are the physical factors that affect flood risk?
Prolonged rainfall.
Soil becomes saturated after prolonged rainfall. This leads to an increase in surface run-off as rainfall can no longer infiltrate the soil. This leads to more water entering the river channel, increasing the likelihood of flooding.
Heavy rainfall
Heavy rainfall can result in water arriving too quickly to infiltrate the soil. This increases surface run-off, leading water to reach the river channel quicker, resulting in a greater risk of flooding.
Impermeable surfaces such as clay and granite do not allow infiltration, leading to greater surface run-off. The risk of flooding increases as water reaches the river channel quickly, increasing discharge and the risk of flooding.
Water can move swiftly downhill in regions with steep terrain, such as mountains. The steeper the slope, the more rapid the water flow into a river channel, increasing the risk of flooding.
What are the human factors that affect flood risk?
Urban development affects water movement as well. Hard, non-absorbent surfaces like concrete, asphalt, and drainage systems can speed up water flow to rivers. Plus, the scarcity of greenery means less rainwater is absorbed or evaporated. Drains also increase water flow into surrounding rivers, increasing the flood risk.
Agricultural practices can influence how water moves. For instance, fields without crops, especially during winter when soils are wet, can quickly channel water. Also, ploughing down slopes can form small channels that accelerate water flow directly to rivers.
Deforestation
Vegetation, including trees, intercepts rainfall, slowing its movement. Some of this water is stored before evaporation returns moisture to the atmosphere. Trees also absorb water from the soil, allowing greater infiltration into the soil and reducing surface run-off. When vegetation is removed, infiltration and interception are reduced and surface run-off increases. Without trees, more water flows directly to rivers, raising the potential for flooding. This leads to a greater risk of flooding as more water reaches the river channel.
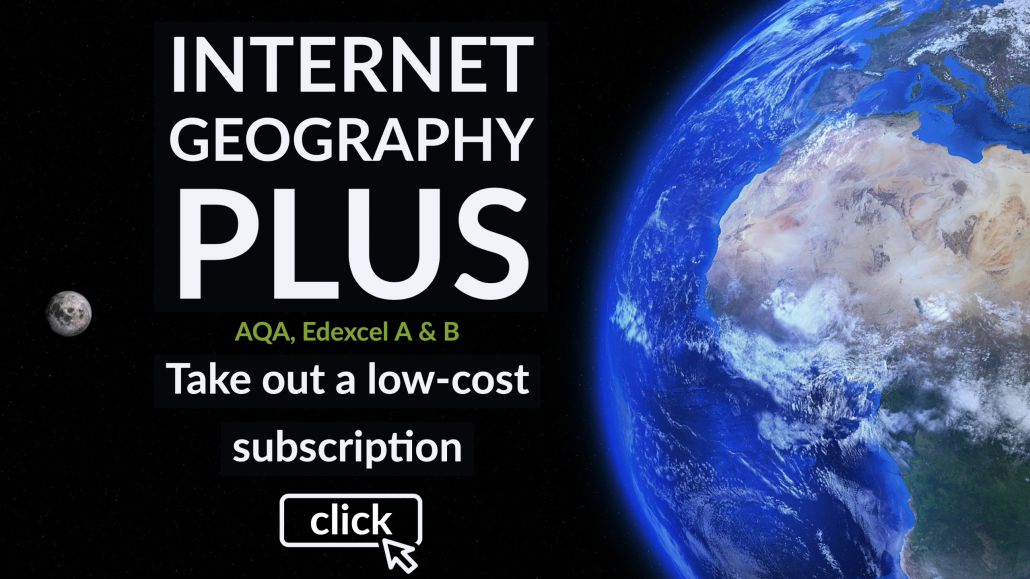
Premium Resources
Please support internet geography.
If you've found the resources on this page useful please consider making a secure donation via PayPal to support the development of the site. The site is self-funded and your support is really appreciated.
Related Topics
Use the images below to explore related GeoTopics.
Human activities in river landscapes
Topic home, how does flooding affect humans and the environment, share this:.
- Click to share on Twitter (Opens in new window)
- Click to share on Facebook (Opens in new window)
- Click to share on Pinterest (Opens in new window)
- Click to email a link to a friend (Opens in new window)
- Click to share on WhatsApp (Opens in new window)
- Click to print (Opens in new window)
If you've found the resources on this site useful please consider making a secure donation via PayPal to support the development of the site. The site is self-funded and your support is really appreciated.
Search Internet Geography
Top posts and pages.

Latest Blog Entries

Pin It on Pinterest
- Click to share
- Print Friendly
Flood and Drought Risk Assessment, Climate Change, and Resilience
- First Online: 30 March 2023
Cite this chapter
- Omar-Darío Cardona 3 , 4 ,
- Gabriel Bernal 4 , 5 &
- María Alejandra Escovar 4
357 Accesses
Work on disaster risk is a challenge due to hazard complexity and interaction between socio-ecological systems. However, potential uses of disaster risk assessment include land-use planning, infrastructure development prioritization, and insurance measures, which can be developed in local, subnational, or national scales by public or private initiatives to build resilience and face the uncertain impacts of climate change. This chapter presents the advantages of using probabilistic risk assessments to assess the potential losses of floods and droughts for crop production and the built environment. Two case studies show that probabilistic flood and drought risk assessments are robust and flexible tools to estimate risk and compare the impacts of hazard control and vulnerability reduction initiatives to ensure risk reduction and resilience.
This is a preview of subscription content, log in via an institution to check access.
Access this chapter
- Available as PDF
- Read on any device
- Instant download
- Own it forever
- Available as EPUB and PDF
- Compact, lightweight edition
- Free shipping worldwide - see info
- Durable hardcover edition
- Dispatched in 3 to 5 business days
Tax calculation will be finalised at checkout
Purchases are for personal use only
Institutional subscriptions
Aguilera, M. (2004). La Mojana: riqueza natural y potencial económico. Documentos de Trabajo Sobre Economía Regional , 48 .
Google Scholar
Banimahd, S. A., & Khalili, D. (2013). Factors influencing Markov chains predictability characteristics, utilizing SPI, RDI, EDI and SPEI drought indices in different climatic zones. Water Resources Management, 27 (11), 3911–3928. https://doi.org/10.1007/s11269-013-0387-z
Article Google Scholar
Berman, I. (2010). Amphibious territories. Achitectural Design, 80 (3), 66–73.
Bernal, G. A. (2018). CAPRA GRM .
Cardona, O. D. (1989). Enfoque metodológico para la evaluación de la amenaza, la vulnerabilidad y el riesgo sísmico. Revista de Ingeniería Sísmica, 37 , 31–63.
Cardona, O. D., Carreño, M., & Barbat, A. (2007). Urban seismic risk evaluation: A holistic approach. Natural Hazards, 40 , 137–172. https://doi.org/10.1007/s11069-006-0008-8
Cardona, O. D., Ordaz, M., Reinoso, E., Yamin, L., & Barbat, A. (2012). CAPRA – Comprehensive approach to probabilistic risk assessment: international initiative for risk management effectiveness. In 15th world conference on earthquake engineering .
Cardona, O. D., Bernal, G. A., Zuloaga, D., Escovar, M. A., & Olaya, J. C. (2017). Modelación probabilista de inundaciones en La Mojana .
Cardona, O. D., Bernal, G., Escovar, M. A., Villegas, C., & González, D. (2018). Perfil de riesgo por sequía e inundación de Uruguay – Estimación del valor expuesto y modelación de la vulnerabilidad . Consorcio INGENIAR – CIMNE.
Eiser, J. R., Bostrom, A., Burton, I., Johnston, D. M., Mcclure, J., Paton, D., et al. (2012). Risk interpretation and action: A conceptual framework for responses to natural hazards. International Journal of Disaster Risk Reduction, 1 , 5–16. https://doi.org/10.1016/j.ijdrr.2012.05.002
Eslamian, S., Reyhani, M. N., & Syme, G. (2019). building socio-hydrological resilience: From theory to practice. Journal of Hydrology, 575 , 930–932.
IPCC. (2012). Managing the risks of extreme events and disasters to advance climate change adaptation: A special report of Working Groups I and II of the Intergovernmental Panel on Climate Change . Cambridge University Press.
IPCC. (2014). Climate change 2014: Synthesis report. Contribution of Working Groups I, II and III to the Fifth Assessment Report of the Intergovernmental Panel on Climate Change (Core Writing Team, R. K. Pachauri, & L. A. Meyer, Eds.). IPCC.
NCAR, & UCAR. (2016). CCSM4.0 public release . Retrieved from http://www.cesm.ucar.edu/models/ccsm4.0/
Ordaz, M. (2000). Metodología para la Evaluación del Riesgo Sísmico Enfocada a la Gerencia de Seguros por Terremoto . Universidad Nacional Autónoma de México.
PNUD. (2019). USD117 Million will be invested in environmental project Mojana: Climate and life . Retrieved from http://www.co.undp.org/content/colombia/es/home/presscenter/pressreleases/2019/02/MojanaClimateAndLife.html
Rougier, J. C. (2011). Quantifying hazard losses. Risk and Uncertainty Assessment for Natural Hazards, 9781107006195 (2003), 19–39. https://doi.org/10.1017/CBO9781139047562.003
Tsakiris, G., Pangalou, D., & Vangelis, H. (2007). Regional drought assessment based on the Reconnaissance Drought Index (RDI). Water Resources Management, 21 (5), 821–833. https://doi.org/10.1007/s11269-006-9105-4
UNISDR. (2015, August). Proposed updated terminology on disaster risk reduction: A technical review (pp. 1–31).
Velásquez, C., Cardona, O. D., Mora, M. G., Yamin, L., Carreño, M., & Barbat, A. (2014). Hybrid loss exceedance curve (HLEC) for disaster risk assessment. Natural Hazards, 72 , 455–479.
Download references
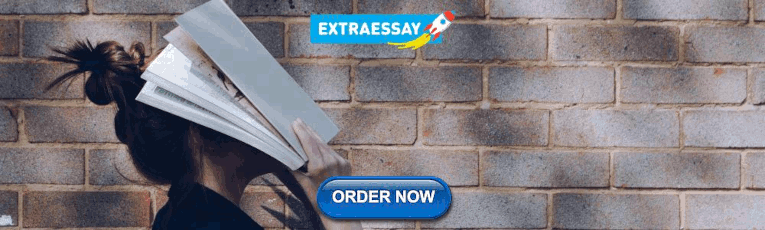
Author information
Authors and affiliations.
Instituto de Estudios Ambientales, Universidad Nacional de Colombia Sede Manizales, Manizales, Colombia
Omar-Darío Cardona
INGENIAR Risk Intelligence, Bogotá, Colombia
Omar-Darío Cardona, Gabriel Bernal & María Alejandra Escovar
Departamento de Ingeniería Civil y Agrícola, Universidad Nacional de Colombia Sede Bogotá, Bogotá, Colombia
Gabriel Bernal
You can also search for this author in PubMed Google Scholar
Corresponding author
Correspondence to Omar-Darío Cardona .
Editor information
Editors and affiliations.
Department of Water Science and Engineering, College of Agriculture, Center of Excellence in Risk Management and Natural Hazards Isfahan University of Technology, Isfahan, Iran
Saeid Eslamian
Department of Bioresource Engineering, McGill University, Montréal, QC, Canada
Faezeh Eslamian
Rights and permissions
Reprints and permissions
Copyright information
© 2023 Springer Nature Switzerland AG
About this chapter
Cardona, OD., Bernal, G., Escovar, M.A. (2023). Flood and Drought Risk Assessment, Climate Change, and Resilience. In: Eslamian, S., Eslamian, F. (eds) Disaster Risk Reduction for Resilience. Springer, Cham. https://doi.org/10.1007/978-3-031-22112-5_9
Download citation
DOI : https://doi.org/10.1007/978-3-031-22112-5_9
Published : 30 March 2023
Publisher Name : Springer, Cham
Print ISBN : 978-3-031-22111-8
Online ISBN : 978-3-031-22112-5
eBook Packages : Earth and Environmental Science Earth and Environmental Science (R0)
Share this chapter
Anyone you share the following link with will be able to read this content:
Sorry, a shareable link is not currently available for this article.
Provided by the Springer Nature SharedIt content-sharing initiative
- Publish with us
Policies and ethics
- Find a journal
- Track your research
Climate Change, Development and Disaster Risk Reduction Essay
Introduction, current approach to climate change, politics, institutions and climate change, future projections for climate change, expected effects of climate change, dealing with climate change.
The climatic conditions of the earth have changed significantly over the centuries. This can be noticed from the geological evidence of changes in sea levels and ice ages. The patterns of human activity over the centuries also give good evidence of climate change.
It is unclear what caused the climate changes in the past, but scientists have listed possible causes like volcanic eruptions, the sun’s effects, and changes in ocean currents (Pelling 2011 p. 17). The evidence of climate change at present is mainly seen in the sharp increase in global temperatures over the past few decades.
The Intergovernmental Panel on Climate Change Fourth Assessment Report arrived at the conclusion that climate change was happening at present. Atmospheric and oceanic temperatures have reached record high levels that have never been witnessed in the last 500 years and most likely for over 1000 years (IPPC 2008 p. 34).
The effects of climate change can be seen in many areas, with one of the most common pointers being the increased frequency of natural disasters. Industrialization and deforestation increase the levels greenhouse gases in the earth’s atmosphere, and this has consequently led to an increase in droughts, floods, hurricanes and other natural disasters.
A look at the statistics indicates that climate change is a major issue that requires a serious approach in order to mitigate its effects. For the period from 1995 to 2004, disasters caused 890,000 deaths and affected a total of 2.5 billion people, with losses estimated at US$ 570 billion (IPCC 2008 p. 46). The number of disasters recorded almost doubled for the period running from 1995 to 2005, and this is cause for major concern.
Other plausible explanations for this increase include population growth and the increase in the number of climatic disasters of a lower magnitude. However, the increased cases of droughts, storms, and very high rainfalls in different places are indicative of the culmination of the effects of climate change, and major disasters are yet to follow in the future (Schipper & Pelling 2006, p. 117).
Some positive statistics show that there are now fewer people dying as a result of disasters as compared to previous centuries. This is particularly so for widespread flooding and drought, which have in the past claimed hundreds of thousands of human lives.
This reduction can be attributed to improved systems for monitoring climatic patterns, providing early warning of impending disaster and general preparedness (Sietz & Boschutz 2011 p. 82). The responses of governments and international organizations through measures like evacuations and food aid have also helped save a lot of lives.
However, this trend could be on the reverse since the past few decades have seen a rise in the baseline level of deaths, which can be attributed to a growing population with a majority of the poor living in disaster prone areas. It is the poor people that feel the full impact of disasters and other effects of climate change.
Any progress that has been made towards poverty reduction over the years can be undone due to the damages caused by these disasters (Pachuari 2004 p. 137).
Many people in the population expect their respective governments to shield them from the risks of disaster at all costs (Wilkinson 2012 p. 156). They do not seriously consider the risks that they may be putting themselves in from the choices they make, especially concerning where they settle and the socio-economic activities that they engage in.
Experts have proposed an approach that decreases the chances of the population suffering impending disasters, regardless of whether they are related to climate change or not.
This idea of disaster risk reduction or adaptation can be implemented in several ways. These include public awareness programs, early warning systems, and environmental protection. These efforts also require serious political commitment and administrative input from respective governments.
Disaster risk reduction and climate change are closely related, yet they are usually discussed under different policy spheres at both national and international forums. In recent years, those concerned with disaster reduction have actively contributed to discussions on climate change, and have proposed concepts for adaptation.
There have been efforts to combine the efforts towards disaster reduction and climate change. There are several UN processes geared towards risk reduction. They include bodies like the United Nations Office of the High Representative for the Least Developed Countries, Landlocked Developing Countries and Small Island Developing States (UNOHRLLS) and the United Nations Economic and Social Council (ECOSOC).
The Small Island Developing States have already recognized the need to reduce the risks of climate change, and have come up with the Mauritius Strategy on sustainable development. The World Trade Organization (WTO) is also helping poor countries that depend on agriculture how to reduce risk and adapt to climate change.
Experts emphasize that the most of the efforts towards reducing climate change and disaster risks should be made at community and state levels. This calls for an integrated approach that unites the efforts of all the concerned sectors under a common framework. Some of these sectors include education, infrastructure, health, and environment.
It has been noted that most of the disaster risk reduction efforts in many countries are handled by the armed forces or emergency management bodies that usually have few connections with other concerned ministries like environment, finance, or economic planning. Many countries are now looking at how to integrate these efforts for better coordination and collaboration between all the major stakeholders (Willis 2005 p. 175).
The public needs to be actively engaged in disaster reduction efforts to complement the efforts by the government and international bodies. The private sector also has a lot of expertise when it comes to disaster management and risk reduction, and their in put should be sought to boost the efforts that are already in place.
Climate change is now the leading cause of many disasters happening all over the world. As a result, the efforts at mitigating the effects of climate change are closely related to disaster risk reduction, and the two must be approached by combining efforts.
Experts are now using computer-based models to try to predict future climate patterns. These models incorporate important factors that affect the atmosphere and the oceans, like the projected increase in greenhouse gases due to socio-economic activities. The results released by the IPCC based on the computer models paint a very grim picture.
Surface air temperatures for the globe are expected to increase by 1.1-6.4 degrees Celsius while seas levels are expected to rise by 18 to 59 cm by the year 2100 (IPCC 2008 p. 58). The acidity of the oceans will increase while the planet will experience heat waves and high precipitation events will become more common. The high precipitation will mainly be seen at higher altitudes while the subtropical areas will see less rainfall.
The continuous rising of tropical sea surface temperatures will cause the tropical cyclones, like typhoons and hurricanes, to become more intense. This will be seen in the higher peak wind speeds and more accompanying precipitation (citation).
Melt water from the top of the mountains supply more than one sixth of the planet’s population with their water needs. These are people living mainly in the dry tropics and mid latitudes, and they are likely to experience a significant reduction in water supply by the middle of the century. There will be higher precipitation in some areas and this is most likely to result in more floods.
The mid and high altitude areas may initially benefit from higher agricultural output due to the increased precipitation. However, in the lower altitude areas, crop production will be heavily affected due to the increases in temperature and frequency of droughts and floods. There will be more people facing hunger and this will cause displacement and migration of large populations.
Those societies, settlements, and industries located at the coastal areas and river flood plains face the highest risks from the effects of climate change. Also under threat are those countries whose economies are heavily dependent on climate-sensitive resources.
The extreme weather patterns have become a common occurrence and with increased intensity, and the economic and social costs of damages resulting from these events will increase. This is more so for places undergoing rapid urbanization. The negative impact of climate change on health is a major concern.
Heat waves, storms, droughts, floods, and fires are likely to cause more injuries and deaths. Other threats to health will come from malaria, diarrhea, and malnutrition. The projects aimed at improving public health especially in the poor countries will suffer a huge setback as a result of disasters (Mehta 2000 p. 89).
Climate change is expected to affect all countries, but the nastiest effects will be experienced by people in poor countries and the poor segment in developed countries. The poor have a tendency to live in high-risk areas like flood plains, yet they do not have properly built houses.
Their economic activities are also dependent on climate, like agriculture, and they have no capacity to cope with the effects of climate change (Madan 2010 p. 67). This means that the low incomes of the poor are likely to fall further, and the rates of illness and death will increase in many developing countries.
Africa already suffers from numerous problems like endemic poverty, armed conflicts, and other disasters yet it lacks the institutional capacity to deal with these situations. This makes the continent extremely vulnerable to the effects of climate change. Drought is set to continue being a major problem in Africa, and it has been shown that sub-Saharan Africa has become drier during the 20 th century.
Agricultural production and water supply are expected to reduce significantly, with some projections putting a reduction in agricultural yields by up to 50% by the year 2020 (Low 2005 p. 248). Asia has been keen on sustainable development but this is set to come under strain as climate change puts more pressure on natural resources that are already under threat from urbanization and industrialization.
The world has slowly awaken to the fact that climate change is a major concern, and countries are now trying to discuss and negotiate ways of dealing with the problem, mainly under the United Nations Framework Convention on Climate Change (UNFCCC).
It has been widely accepted that greenhouse emissions arising from human activity are the main problem, and focus is now on how to reduce these emissions (Levine, Ludi & Jones 2011, p. 46). There is a lot of contention on how to go about reducing greenhouse emissions, as it calls for radical changes that will affect the society in a major way.
The use of fossil fuels, industries, urban development, and general land use all need to be changed drastically and this is likely to affect many people in a huge way hence the resistance. The efforts at reducing greenhouse emissions are referred to as “mitigation”.
Some of the proposed mitigation actions include switching to renewable forms of energy, development of low-energy technologies and reducing reliance on energy-intensive products. It also involves environmental management and conservation efforts targeting forests and soils. The second plan of action in response to climate change involves finding ways of managing its impacts.
The amounts of greenhouse gases that have already accumulated in the atmosphere mean that the world cannot escape the impacts of climate change. This is reinforced by the continued emissions before the mitigation efforts take full effect.
It is widely accepted that the impacts of climate change are coming or are already here, and some effort is being put towards lessening these impacts. These efforts towards coping with climate change are referred to as “adaptation”.
Proposed adaptation efforts include early warning systems, better building designs, improved agricultural practices, and putting in place social safety nets for the poor. Adaptation and mitigation efforts should go hand in hand for complimentary effect (Jones et al. 2010 p. 79).
Geological and anthropological evidence indicate that the earth has experienced climate change before. It is difficult to point out the real reasons behind climate change in the past. However, climate change is happening now, and much of it is due to human activity.
Both atmospheric and oceanic temperatures have reached record highs that have not been experienced for hundreds of years. The effects of climate change are already being felt, especially due to the increased frequency of disasters all over the world. These changes have mostly affected poor people living in disaster prone areas (Baker 2012 p. 28).
The most common effects are extreme weather conditions like heavy rainfall that results in widespread floods, and prolonged drought that causes famine and denies the poor a source of livelihood through agriculture. There have been efforts towards addressing climate change as the world continues to witness its devastating effects.
However, there seems to be several hindering factors, mainly at local and national levels (Dasgupta & Baschieri 2010 p. 56). Most countries lack the capacities and institutional frameworks to deal with climate change, and there has also been slow reaction from governments yet they are expected to play a significant role in the whole process.
Efforts at addressing climate change revolve around mitigation actions aimed at reducing greenhouse emissions, and adaptation actions meant to reduce the impacts of climate change.
Mitigation requires some drastic measures that are bound to affect many people in a significant way, and this has caused a lot of debate. As for adaptation, it has come to be accepted that climate change is already causing problems, with more to come so people are trying to find ways of lessening the impact.
Baker, J L 2012, Climate change, disaster risk, and the urban poor: Cities building resilience for a changing world , World Bank, Washington, D.C.
Dasgupta, A & Baschieri, A 2010, ‘Vulnerability to Climate Change in Rural Ghana: Mainstreaming Climate Change in Poverty-Reduction Strategies’, Journal of International Development , vol. 954 no.748, pp. 803-820
Intergovernmental Panel on Climate Change 2008, Climate change 2007: impacts, adaptation and vulnerability : Working Group II contribution to the Fourth Assessment Report of the IPCC Intergovernmental Panel on Climate Change , IPCC Secretariat, Geneva.
Jones, L., Jaspars, S., Pavanello, S., Ludi, E., Slater, R., Arnall, A., Grist, N and Mtisi, S 2010, Responding to a changing climate: Exploring how disaster risk reduction, social protection and livelihoods approaches promote features of adaptive capacity , Overseas Development Institute, London.
Levine, S., Ludi, E. and Jones, L 2011, Rethinking Support for Adaptive Capacity to Climate Change The Role of Development Interventions , Overseas Development Institute, London
Low, S 2005, Climate change and Africa , Cambridge Univ. Press, Cambridge [u.a.],
Madan K 2010, Natural and anthropogenic disasters: vulnerability, preparedness and Mitigation , Springer, Dordrecht
Mehta, l 2000, ‘Environmental Governance in an Uncertain World’, IDS Bulletin , vol. 32 no. 4, pp. 1-15
Pachauri, R 2004, ‘Climate Change and Its Implications for Development’, IDS Bulletin , vol. 35 no. 3, pp. 11-14.
Pelling, M 2011, ‘Conclusion: adapting with climate change’, Adaptation to climate change: from resilience to transformation , Routledge: New York,
Schipper, L and Pelling, M 2006, ‘Disaster risk, climate change and international development: Scopes for, and challenges to, integration’, Disasters , vol. 30 no. 1, pp. 19-38.
Sietz, D and Boschutz, M 2011, ‘Mainstreaming climate adaptation into development assistance: rationale, institutional barriers and opportunities in Mozambique, Environmental Science & Policy , vol. 14 no. 4, pp 493-502.
Wilkinson, E 2012, Transforming disaster risk management: a political economy approach , Overseas Development Institute, London.
Willis, K 2005, Theories and Practices of Development , Routledge, Abingdon.
- Chicago (A-D)
- Chicago (N-B)
IvyPanda. (2019, April 11). Climate Change, Development and Disaster Risk Reduction. https://ivypanda.com/essays/climate-change-development-and-disaster-risk-reduction/
"Climate Change, Development and Disaster Risk Reduction." IvyPanda , 11 Apr. 2019, ivypanda.com/essays/climate-change-development-and-disaster-risk-reduction/.
IvyPanda . (2019) 'Climate Change, Development and Disaster Risk Reduction'. 11 April.
IvyPanda . 2019. "Climate Change, Development and Disaster Risk Reduction." April 11, 2019. https://ivypanda.com/essays/climate-change-development-and-disaster-risk-reduction/.
1. IvyPanda . "Climate Change, Development and Disaster Risk Reduction." April 11, 2019. https://ivypanda.com/essays/climate-change-development-and-disaster-risk-reduction/.
Bibliography
IvyPanda . "Climate Change, Development and Disaster Risk Reduction." April 11, 2019. https://ivypanda.com/essays/climate-change-development-and-disaster-risk-reduction/.
- Flooding and Mitigation Measures
- Victim Precipitation Ideology
- Climate Change: Floods in Queensland Australia
- Global Warming: Justing Gillis Discussing Studies on Climate Change
- Environmental Defense Fund
- Ecosystems: Biodiversity and Habitat Loss
- Spin, Science and Climate Change
- Greenhouse Gases Prediction
Flood Essay for Students and Children
500+ words essay on flood.
Flood is one of the most dangerous natural disasters. It happens when excessive water is collected in any area. It usually happens due to heavy rainfall. India is highly prone to flood. There are many regions in the country that face this natural disaster because of the overflowing of rivers. Moreover, it also happens because of the melting of snow. Another reason for floods is when the dam breaks down. If we look at the coastal areas, the hurricanes and tsunamis are held responsible for causing floods. In this essay on flood, we will see the prevention and after-affect of flood.

In other words, whatever the cause may be, it is equally dangerous. It has a lot of harmful consequences. Flood damages the living conditions and it takes a lot of time to recover from this disaster. Therefore, the consequences of floods must be known and steps must be taken to prevent it.
After-effects of Flood
Floods interrupt with the day to day functioning of the affected area. The severe floods sometimes cause mass destruction. A lot of people and animals lose their lives due to floods. Several others are injured. Floods also bring a rise in diseases. The stagnant water attracts mosquitoes causing malaria , dengue, and more illnesses.
Furthermore, people face power cuts due to the danger of electrocution. They also have to face expensive pricing. As the supply of food and goods gets limited, the prices naturally grow higher. This creates a big problem for the common man.
Most importantly, the whole country faces economic loss. The resources needed to rescue people and tackle this disaster demands a hefty amount. Plus, the citizens lose their houses and cars which they worked all their lives for.
Subsequently, floods also hamper the environment. It causes soil erosion and this degrades the quality of the soil. We lose out on fertile soil. Similarly, floods also damage flora and fauna. They damage crops and displace trees. Thus, the measure should be taken to avoid these grave consequences.
Get the huge list of more than 500 Essay Topics and Ideas
Ways to Prevent flood
The government and citizens must work together to formulate ways to prevent floods. Proper awareness must be spread about the steps to take when floods occur. Warning systems must be set up so people get sufficient time to save themselves. In addition, areas that are more likely to have floods must have tall buildings above the flood level.

Other than that, dams must be constructed strongly. The use of cheap materials causes dams to break. The government must ensure there is a quality building of dams to prevent floods.
In short, we cannot prevent natural causes like rain and the melting of glaciers. However, we can stop the manmade causes like breaking of dams, poor drainage system, installing warning systems and more. We should take inspiration from countries like Singapore that never experience floods despite having heavy rainfall for most time of the year.
FAQ on Flood Essay
Q.1 what are the consequences of a flood.
A.1 Floods cause immense destruction. They are responsible for the loss of human and animal lives. People lose their homes and cars in floods. They also cause soil erosion and uproot of trees.
Q.2 How can we prevent floods?
A.2 Governments must take up certain measures to prevent floods. We can install flood warning systems. Make people aware of what to do in times of flood. Moreover, we can also build a proper drainage system that will ensure no waterlogging.
Customize your course in 30 seconds
Which class are you in.

- Travelling Essay
- Picnic Essay
- Our Country Essay
- My Parents Essay
- Essay on Favourite Personality
- Essay on Memorable Day of My Life
- Essay on Knowledge is Power
- Essay on Gurpurab
- Essay on My Favourite Season
- Essay on Types of Sports
Leave a Reply Cancel reply
Your email address will not be published. Required fields are marked *
Download the App
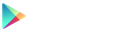

Essay on Importance Of Disaster Risk Reduction
Students are often asked to write an essay on Importance Of Disaster Risk Reduction in their schools and colleges. And if you’re also looking for the same, we have created 100-word, 250-word, and 500-word essays on the topic.
Let’s take a look…
100 Words Essay on Importance Of Disaster Risk Reduction
What is disaster risk reduction.
Disaster Risk Reduction (DRR) means preparing for disasters before they happen. It’s like studying for a test in advance so you’re ready. DRR helps people stay safe during events like earthquakes, floods, or storms. It’s important because it saves lives and protects our homes and schools.
Why DRR Matters
When we reduce disaster risks, we make sure fewer people get hurt. It’s like wearing a helmet when riding a bike. By being prepared, communities don’t lose as much and can bounce back quicker after disasters.
DRR in Action
DRR includes teaching people what to do when disasters strike and building stronger houses. It also means keeping nature healthy, like forests that can stop floods. When we all work on DRR, we make our world a safer place.
250 Words Essay on Importance Of Disaster Risk Reduction
Disaster Risk Reduction, or DRR, means taking steps to make sure that natural events like earthquakes, floods, and storms cause less harm to people and places. It’s like putting on a helmet before riding a bike to protect your head, just in case you fall.
Why is DRR Important?
Firstly, DRR saves lives. By preparing for disasters before they happen, fewer people get hurt or lose their lives. Think of it as learning to swim to stay safe in water. If a flood comes, knowing what to do can keep you and your family safe.
Secondly, it protects our homes and schools. Strong buildings and safe roads mean that even if a disaster happens, the places where we live and learn don’t get destroyed.
DRR Helps Everyone
DRR is not just for adults; it’s for everyone, including kids. When children know about DRR, they can help their friends and family stay safe. It’s like knowing the rules of a game so that everyone can play without getting hurt.
DRR Saves Money
Finally, DRR saves money. Fixing things after a disaster can be very expensive. It’s cheaper to make things strong and safe from the start. It’s like fixing a small crack in a wall before it becomes a big hole that’s hard and costly to repair.
In conclusion, DRR is very important because it keeps us safe, protects our homes and schools, involves everyone, and saves money. Just like wearing a helmet or learning to swim, being ready for disasters is a smart choice.
500 Words Essay on Importance Of Disaster Risk Reduction
Understanding disaster risk reduction.
Disaster Risk Reduction, or DRR, is a way of making sure that when natural events like earthquakes or floods happen, they cause as little harm as possible to people, their homes, and their way of life. It’s like putting on a helmet before riding a bike; it doesn’t stop accidents from happening, but it can make them less painful.
Why DRR is Important
Imagine living in a place where a big storm could come at any time. If nothing is done to prepare for it, the storm could destroy houses, schools, and hospitals, leaving many people hurt or without a place to live. But if the town has strong buildings, emergency plans, and people know what to do when the storm comes, then the damage can be much less. This is the main goal of DRR: to make sure that when nature gets rough, we can stand strong and bounce back quickly.
Protecting Lives and Homes
The first and most important reason for DRR is to save lives. When we take steps to reduce disaster risks, like building houses that can stand up to strong winds or teaching people how to safely leave a flooding area, we are making sure that fewer people get hurt or lose their lives when disasters strike.
Keeping Schools and Hospitals Safe
Schools and hospitals are very important because they help us learn and stay healthy. DRR makes sure that these places are built in a way that they can keep working even after a disaster. This means that after a disaster, kids can go back to school quickly, and people who are hurt can get the help they need.
Helping Communities Recover Faster
When a disaster happens, it can take a long time for a place to get back to normal. But with good DRR, communities can fix things faster and with less cost. This is because they already have plans and tools ready to help rebuild homes and businesses, and they know how to work together to get things done.
DRR is Good for the Economy
Disasters can cost a lot of money because they can destroy shops, farms, and other ways people make a living. By reducing the damage from disasters, DRR helps people keep their jobs and businesses, which is good for the whole country’s money situation.
Everyone Plays a Part
DRR isn’t just for the government or big organizations; it’s something everyone can help with. By learning about the risks in your area and what to do in an emergency, you can be a part of keeping your family and friends safe.
In conclusion, Disaster Risk Reduction is very important because it helps protect people, their homes, and the places they go to learn and get better when they are sick. It also makes it easier for towns and cities to fix things after a disaster and helps keep the economy stable. By working together to reduce risks, we can make sure that when nature shows its power, we are ready and can keep the harm to a minimum.
That’s it! I hope the essay helped you.
If you’re looking for more, here are essays on other interesting topics:
- Essay on Importance Of Digital Marketing
- Essay on Importance Of Dance
- Essay on Importance Of Daily Routine
Apart from these, you can look at all the essays by clicking here .
Happy studying!
Leave a Reply Cancel reply
Your email address will not be published. Required fields are marked *
Save my name, email, and website in this browser for the next time I comment.

- Research article
- Open access
- Published: 07 May 2024
Floods and cause-specific mortality in the UK: a nested case-control study
- Danijela Gasevic 1 ,
- Zhengyu Yang 1 ,
- Guowei Zhou 2 ,
- Yan Zhang 2 ,
- Jiangning Song 3 ,
- Hong Liu 2 na1 ,
- Shanshan Li 2 na1 &
- Yuming Guo ORCID: orcid.org/0000-0002-1766-6592 2 na1
BMC Medicine volume 22 , Article number: 188 ( 2024 ) Cite this article
688 Accesses
18 Altmetric
Metrics details
Floods are the most frequent weather-related disaster, causing significant health impacts worldwide. Limited studies have examined the long-term consequences of flooding exposure.
Flood data were retrieved from the Dartmouth Flood Observatory and linked with health data from 499,487 UK Biobank participants. To calculate the annual cumulative flooding exposure, we multiplied the duration and severity of each flood event and then summed these values for each year. We conducted a nested case-control analysis to evaluate the long-term effect of flooding exposure on all-cause and cause-specific mortality. Each case was matched with eight controls. Flooding exposure was modelled using a distributed lag non-linear model to capture its nonlinear and lagged effects.
The risk of all-cause mortality increased by 6.7% (odds ratio (OR): 1.067, 95% confidence interval (CI): 1.063–1.071) for every unit increase in flood index after confounders had been controlled for. The mortality risk from neurological and mental diseases was negligible in the current year, but strongest in the lag years 3 and 4. By contrast, the risk of mortality from suicide was the strongest in the current year (OR: 1.018, 95% CI: 1.008–1.028), and attenuated to lag year 5. Participants with higher levels of education and household income had a higher estimated risk of death from most causes whereas the risk of suicide-related mortality was higher among participants who were obese, had lower household income, engaged in less physical activity, were non-moderate alcohol consumers, and those living in more deprived areas.
Conclusions
Long-term exposure to floods is associated with an increased risk of mortality. The health consequences of flooding exposure would vary across different periods after the event, with different profiles of vulnerable populations identified for different causes of death. These findings contribute to a better understanding of the long-term impacts of flooding exposure.
Peer Review reports
Floods are the most frequent type of weather-related disaster, accounting for about 47% of all weather-related disasters from 1995 to 2015 [ 1 , 2 ]. Between 1995 and 2015, more than 2.3 billion people were affected by flood disasters, with over 157 thousand people dying directly as a result of floods [ 3 ]. In recent years, many intense urban flooding events have been recorded in the UK, resulting in loss of lives, damages to personal property and public health infrastructure, and disruption to vital services such as water, communications, energy, and public transport [ 4 , 5 , 6 , 7 , 8 , 9 ]. Approximately 1.9 million people across the UK are at risk of floods, and this number will double as early as the 2050s [ 10 ].
In addition to immediate fatalities due to drowning and acute trauma [ 11 ], floods can also cause short- (lasting days or weeks) or medium-health impacts (several weeks or months), including the spread of water- and vector-borne diseases, such as cholera, typhoid, or malaria; injuries during evacuations and disaster clean-up; and exposure to chemical hazards [ 1 , 12 ]. Non-communicable diseases (e.g. cardiovascular disease, neoplasms, chronic respiratory diseases, and diabetes) which need prolonged treatment and care can be exacerbated after floods due to a disruption in care, treatment, medication, supplies, equipment, and overcrowding in shelters [ 13 , 14 , 15 , 16 , 17 , 18 ]. Mental health issues may arise from stressors caused by floods (e.g. property damage, financial loss, loss of a loved one) and have long-lasting health effects on mortality and morbidity. These long-term health consequences may arise from several pathways, including impairment of the immune system, sleep disturbances, substance abuse, and inadequate self-care [ 19 , 20 , 21 , 22 ].
Despite the severe impacts of floods, there currently is limited epidemiological evidence on the long-term mortality impacts of exposure to floods. To address these gaps in knowledge, we utilized the UK Biobank project, a population-based study with a large sample size, to explore the long-term effects of flooding on mortality. We aimed to estimate the risk of all-cause and seven cause-specific mortality associated with floods and to explore the lag patterns in mortality risk. We also conducted subgroup analyses to identify populations who are potentially more vulnerable to flood-related death.
Study design and study population
We conducted a nested case-control study within a cohort of participants registered with the UK Biobank study. About 0.5 million residents aged between 37 and 73 years were enrolled in the UK Biobank from 2006 to 2010, from 21 assessment centres across England, Wales, and Scotland. The cohort was followed up until the date of death or the study end date (December 31, 2020). We excluded participants lacking longitude and latitude data of residence ( n = 11), participants with missing data on age, sex, and ethnicity ( n = 2775), and those who died in the year of recruitment ( n = 141). A total of 499,487 participants were included (Fig 1 ). All participants in the UK Biobank study provided informed consent. The utilization of the data presented in this paper has been approved by the UK Biobank access committee under UK Biobank application number 55257.
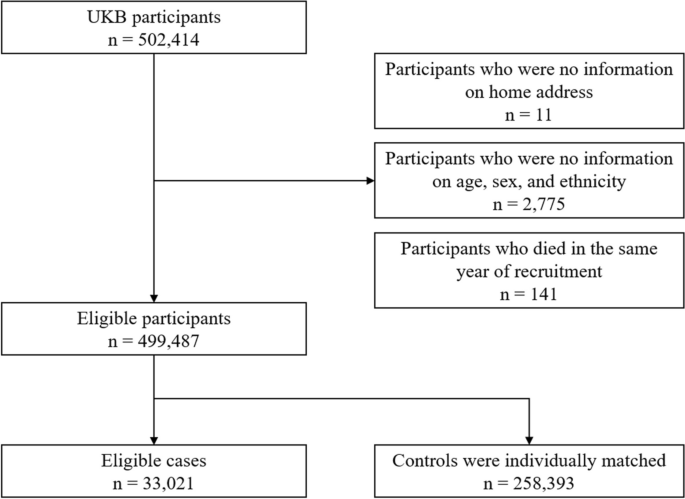
A flow diagram to show participants whose data were used to estimate the association between flooding exposure and mortality
Case-control selection
With the nested case-control design, we matched controls to cases with replacement at the time of the outcome event and assessed exposure retrospectively, from the date of death or end of follow-up. This ensures identical exposure lengths across participants. Using a risk-set sampling method, each case was matched with eight controls randomly selected from study participants who met the matching criteria for age (within 5 years), sex (male and female), and ethnicity (White, Black, Asian or Asian British, mixed, Chinese, and others). The index date for cases corresponded to the date of death; while for controls, it was the date of death of the matched case participant. For twelve case-control sets, eligible controls were less than eight but at least one (Fig 1 ).
Participants were eligible for inclusion as cases for the study if they died during the study period. We defined all-cause mortality and seven cause-specific mortality categories using the International Classification of Diseases, edition 10 (ICD-10), classification as follows: neoplasms, C00–D48; cardiovascular disease, I00–I99; respiratory diseases, J09–J98; digestive disease, K20–K93; neurodegenerative disease, F01–03, G122, G20, G21, G23, G30, G31; mental and behavioural disorders: F00–F90; and suicide: X60–X84, Y10–Y34, Y87.
Flooding exposure
We collected flood data during 2000–2020 from the Dartmouth Flood Observatory (DFO), which is a global catalogue of all flood events with detailed information on start date, end date, centroids, impacted geographic areas, and severities. All documented flood events were sourced from news, government, and instrumental sources and have been validated by satellite observations [ 23 , 24 ]. Participants whose home addresses fall within flood-affected areas were considered as having been exposed to a flood event. To assess the long-term effect of floods, we calculated a cumulative exposure during the study period for each participant. Building on previous research [ 25 , 26 ], we derived the annual cumulative exposure by multiplying the duration and severity of each flood event and summing these values for each year. Our preliminary analyses suggested a weak negative association between flood severity and duration (Pearson coefficient: − 0.03). The severity of each flood event documented in the DFO was classified based on a pre-defined scale, detailed in Additional file 1 : Table S1. For each participant, annual cumulative flooding exposure was calculated using equation ( 1 ):
where \(\mathrm{Flood}\;{\mathrm{index}}_{i,\text{year}=m}\) stands for the cumulative flooding exposure in year \(m\) for participants \(i\) . \({{\text{Duration}}}_{ij}\) and \({{\text{Severity}}}_{ij}\) represent the duration (day) and the severity of the \(j\) th flood event that participant \(i\) experienced in year \(m\) , respectively. If there were no flood events within a given year, a flood index of 0 was recorded.
Meteorological data
We extracted hourly temperature and relative humidity data from the European Centre for Medium-Range Weather Forecasts Reanalysis v5 (ERA-5) reanalysis data set with a spatial resolution of 0.1°×0.1°. We mapped meteorological data to the participant’s geocoded residential address at baseline. Daily meteorological data were calculated by averaging hourly data within each day. Daily temperature and relative humidity were then aggregated into yearly averages.
Baseline data collected by the UK Biobank include demographics, lifestyle factors, socioeconomic status, and anthropometric measurements. We included additional covariates informed by existing literature, beyond those used for matching cases and controls [ 27 , 28 , 29 ]: body mass index (BMI), physical activity, healthy diet score, cigarette smoking, alcohol consumption, educational attainment, average total annual household income before tax, Townsend deprivation index (TDI), overall health rating, and assessment centres. BMI was calculated from objectively measured weight and height as weight over height squared and expressed as kg/m 2 . Physical activity was derived from the International Physical Activity Questionnaire-Short Form (IPAQ-SF) [ 30 ]. Participants were categorized at ‘high’ (≥ 1500 metabolic equivalent (MET)-minutes/week), ‘moderate’ (≥ 600 MET-minutes/week), or ‘low’ levels of physical activity following standardized IPAQ-SF scoring guidance [ 30 ]. Diet score was calculated based on the following dietary factors: vegetable intake ≥ 3 servings/day; fruit intake ≥ 3 servings /day; whole grains ≥ 3 servings/day; refined grains ≤ 1.5 servings/day; fish intake ≥ 2 servings/day; unprocessed red meat intake ≤ 2 servings/week; and processed meat intake ≤ 2 servings/week. Each point was given for each favourable dietary factor, and the suboptimal diet was defined as a diet score < 4. Smoking status was coded into three categories: current, former, and never. Low-risk alcohol consumption was defined as moderate drinking (no more than one drink/day for women and two drinks/day for men; one drink is measured as 8 g ethanol in the UK) on a relatively regular frequency [ 31 ]. Educational attainment was coded in two categories: ‘high’ (college or university degree) or ‘low’ (A/AS levels or equivalent, O levels/GCSEs or equivalent, or none of the above). Annual household income was classified into two groups (< £31,000 and ≥ £31,000). TDI was utilized to define area deprivation level, with participants being classified as either high (TDI above the median) or low [ 32 ]. Self-reported health was categorized as poor, fair, good, and excellent [ 27 ].
Statistical analysis
We performed conditional logistic regression analysis to estimate the risk of mortality associated with per unit increase in flood index. Year-specific flood index was modelled using a distributed lag non-linear model featuring a non-linear exposure-response association and the additional lag-response association, respectively [ 33 , 34 , 35 , 36 ]. The lag-response association refers to how the risk changes over time and provides an estimation of the combined immediate and delayed effects that accumulate throughout the lag period. We first modelled the exposure-response curve with a natural cubic spline with three degrees of freedom. However, the nonlinear analysis indicated an approximately linear relationship (Additional file 1 : Fig. S1). Further, both the Akaike Information Criterion (AIC) and the Bayesian Information Criterion (BIC) favoured the linear model (Additional file 1 : Table S2). Therefore, we applied a linear exposure-response relationship in the formal analysis. The lag-response curve was modelled with a natural cubic spline with three degrees of freedom plus an intercept. The exposure window comprised the 0 to 5 years before the index date. A maximum lag of 5 years was used because the flood-related mortality risk declined to zero by the lag year 5.
Estimates of risk were obtained from the crude model that only included flood (model 1); the multivariate model that additionally controlled for socioeconomic status (education attainment, household income, and deprivation) (model 2); and the full model that additionally adjusted for BMI, physical activity, smoking, alcohol consumption, suboptimal diet, overall health rating, mean temperature, mean relative humidity, and assessment centre which serves as an indicator of the recruitment location for each participant (model 3). All variance inflator factors were less than 1.5, indicating no multicollinearity. Temperature and relative humidity terms were defined as the average annual mean temperature and relative humidity over 6 years (lag 0–5 years) preceding the index date, respectively. Given that the crude model (model 1) did not include any covariates, all participants were retained in the analysis. For models 2 and 3, we excluded participants with any missing data. In sensitivity analyses, we employed multiple imputation to address missing covariate data and assess the robustness of our findings.
We further identified subgroups vulnerable to floods through stratification analyses by age group (≤64 and >65 years), sex, weight status defined according to BMI (≤ 24.9, 25–29.9, ≥ 30), education attainment, household income, physical activity, suboptimal diet, alcohol consumption status, smoking status, and area deprivation level. Results are presented as odds ratios (ORs) and their 95% confidence intervals (95% CIs) per unit increase in flood index. The significance of the difference in results between subgroups was tested using a random-effect meta-regression model.
Sensitivity analysis
We carried out the following sensitivity analyses: (1) Multiple imputation by chained equations was used for the missing values. Five imputed data sets were created, and their results were combined using Rubin’s rules [ 37 ]. (2) Alternative degrees of freedom were used for the lag-response association of flood. (3) Alternative degrees of freedom were used for the non-linear exposure-response relationship of mean temperature and relative humidity. (4) Alternative matching ratios (1:4 and 1:6) were used. (5) Excluding data after 2020 to control for the effect of the COVID-19 pandemic. (6) To capture the variation in flooding impacts within the year preceding mortality, we performed additional analyses with monthly flood index.
Table 1 shows the baseline characteristics of the 33,021 death cases and the 258,393 matched controls. The mean age (± standard deviation (SD)) of participants at study entry was 61.3 (± 6.4) years; 170,549 (58.5%) were male; 281,175 (96.5%) were white. Participants who died were more likely to have a higher BMI and lower household income; were less likely to be university graduates; more likely to smoke; and consumed less fruit and vegetables and more red and processed meat. They were also more likely to rate their overall health as poor and fair. Baseline characteristics of cases and controls with any missing values in covariates are shown in Additional file 1 : Table S3.
The distributions of the flood index and meteorological factors are shown in Table 2 . The annual average flood index across all participants during the study period ranged from 0.0 to 38.3, with a median value of 1.8 (25 th to 75 th percentiles: 0.5 to 3.6). Cases exposed to higher levels of flooding than controls during the 6 years before the end of follow-up (Additional file 1 : Fig. S2). The median annual mean temperature was 10.0 °C (25 th to 75 th percentiles: 9.3°C to 10.7°C) (Table 2 ). The flood index was negatively correlated with mean temperature (Pearson r = − 0.04) but positively correlated with relative humidity (Pearson r = 0.08).
Figure 2 illustrates the estimated cumulative OR of all-cause and cause-specific mortality associated with per unit increase in flood index over lag years 0–5. Per unit increase in flood index was associated with a 9.2% increased risk of all-cause mortality (OR: 1.092, 95% CI: 1.090–1.093) in the crude model. The results remained similar after further adjustment for socio-economic status (OR: 1.090, 95% CI: 1.088–1.091), whereas adjustment for lifestyle factors decreased the strength of the association (OR for fully adjusted model: 1.067, 95% CI: 1.063–1.071). Similar effects were observed for cause-specific mortality after fully adjusting the models, whereby a greater flood index was associated with a greater risk of death from neurodegenerative diseases (OR: 1.068, 95% CI: 1.050–1.087), neoplasm (OR: 1.063, 95% CI: 1.058–1.068), respiratory diseases (OR: 1.062, 95% CI: 1.045–1.080), suicide (OR: 1.052, 95% CI: 1.018–1.088), cardiovascular diseases (OR: 1.051, 95% CI: 1.042–1.059), mental diseases (OR: 1.047, 95% CI: 1.008–1.087), and digestive diseases (OR: 1.031, 95% CI: 1.011–1.052) (Fig. 2, Additional file 1 : Table S4).
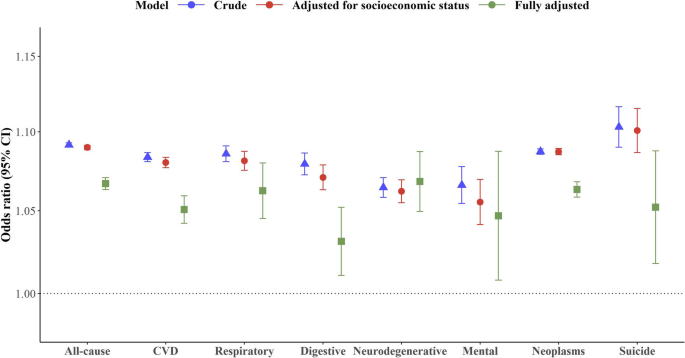
Cumulative odds ratio of all-cause and cause-specific mortality associated with per unit increase in flood index over lag years 0–5. Estimates of risk were obtained from the crude model that only included flood (crude); the multivariate model that additionally controlled for socioeconomic status (education attainment, household income, and deprivation); and the full model that additionally adjusted for BMI, physical activity, smoking, alcohol consumption, suboptimal diet, overall health rating, mean temperature, mean relative humidity, and assessment centre which serves as an indicator of the recruitment location for each participant (fully adjusted). The error bars represent 95% confidence intervals
Figure 3 shows the lag structure in the effects of flooding exposure on all-cause and cause-specific mortality. For all-cause mortality, the magnitude of associations increased from the current year (OR: 1.012, 95% CI: 1.011–1.013) to the lag year 3 (OR: 1.016, 95% CI: 1.015–1.017), and subsequently diminished to zero by lag year 5. For neurodegenerative mortality and mortality due to mental-ill health, the mortality risk was negligible in the current year, but strongest in the lag years 3 and 4. By contrast, the risk of mortality from suicide was the strongest in the current year (OR: 1.018, 95% CI: 1.008–1.028), and attenuated to lag year 5.
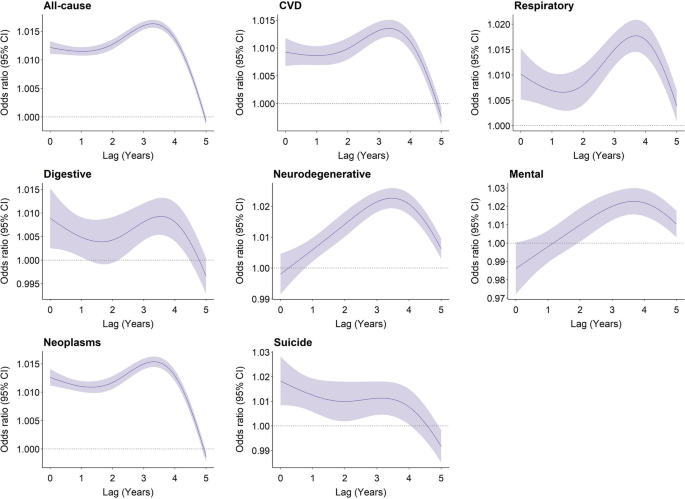
Overall lag structure in effects of flooding exposure on cause-specific mortality. Shaded areas represent 95% confidence intervals for the odds ratio
Subgroup analyses revealed that participants with higher levels of education and household income had a higher estimated risk of death from most causes in association with flooding exposure. Participants aged below 64 and female had a higher estimated risk of death from all-cause mortality, respiratory diseases, and neoplasm, but a lower estimated risk of death from digestive and mental diseases, respectively. The risk of suicide-related mortality in association with flooding exposure was higher among participants who were obese, had lower household income, engaged in less physical activity, were non-moderate alcohol consumers, and had high deprivation levels (Table 3 ).
Our sensitivity analysis suggested that using multiple imputed data did not change study findings (Additional file 1 : Fig. S3). Our results were not dependent on modelling assumptions and remained unaffected by the COVID-19 pandemic (Additional file 1 : Fig. S4–7). Matching ratios of 1:4 and 1:6 revealed a modest increase in the odds ratio for all-cause mortality and neoplasms, while the odds ratio for other causes of death remained unchanged ( Additional file 1 : Fig. S8 ). For all-cause mortality, with per unit increase in monthly flood index, odds ratios over 0–12 months preceding the mortality ranged from 1.005 (95% CI: 1.005–1.006) to 1.018 (95% CI: 1.017–1.018) (Additional file 1 : Table S5).
In this nested case-control study, we observed a significantly increasing risk of mortality associated with floods. The exposure-response curve was linear, with no discernible thresholds. The lag pattern varied across different causes of death. Flooding exposure has a long-lasting impact on neurodegenerative and mental diseases, whereas it has an immediate impact on suicide. Subgroup analyses revealed specific groups of vulnerable populations for flood-related death, which varied according to the cause of death.
Every unit increase in flood index was associated with a 6.7% increase in all-cause mortality risk over the following 6 years. This finding was similar to cause-specific mortality. Although few epidemiological studies have assessed the long-term effect of floods on mortality, our findings are consistent with previous findings of short-term flooding exposure showing increased risk of cholera at lag 0–20 weeks [ 38 ], diarrhoea at lag 0–28 weeks [ 38 ], respiratory infection at lag 3 months [ 39 ], typhoid fever at lag 1 week [ 40 ], malaria at lag 1 year [ 12 ], malnutrition at lag 1 year [ 41 ], and mental disorders at lag 6 months [ 19 ]. One study assessing the effects of flooding on mortality in England and Wales during 1994–2005 suggested a deficit of deaths in the post-flood period [ 42 ]. The inconsistency might result from the underestimation of death number, which can occur when deaths are registered at different places after displacement and a short observation period (one year after flooding exposure) during which the occurrence of death has not been observed. Milojevic et al. reported a slight but non-significant increase in mortality rates following the floods in Bangladesh in the flooded areas compared to non-flooded areas [ 43 ]. The accuracy of their results might be subject to recall bias in exposure assessment, given that exposure to flooding was ascertained from an interview survey four years after the flood event.
The long-term health deterioration resulting from floods could be attributed to mental health disorders driven by financial losses and community or social disruption, especially for those who live in resource-poor countries and communities (e.g. floodplains or non-resistant buildings, lack of warning systems and awareness of flooding hazard) [ 1 , 44 ]. For example, previous studies reported a significant and continued increase in the prevalence of post-traumatic stress disorder (PTSD), stress, anxiety, depression, and even suicide ideation following flooding exposure [ 20 ], which contribute to worse health outcomes. Additionally, among people who were exposed to floods, those who had chronic medical conditions are at higher risk of health deterioration due to potential disruptions in medication and healthcare services. Previous studies have noted that older adults and those receiving long-term care services showed decreased treatment adherence (e.g. interruption of medication and access to physicians) months and even years after the flooding [ 45 , 46 ], which serve to exacerbate or prolong symptoms of existing conditions.
Flooding exposure has a long-lasting impact on neurodegenerative and mental diseases, reaching its peak at 3–4 years post-exposure whereas the highest risk of mortality due to suicide occurs in the year of exposure. This suggests that varying health issues should be given consideration, depending on the stage following flooding exposure. A study in Queensland has noted that direct exposure to flood resulted in an increase in alcohol and tobacco usage half a year after flooding [ 46 ] and use of substances has been associated with an increased risk of suicide attempts in previous studies [ 47 , 48 , 49 , 50 ]. However, social support and compensation coverage have been demonstrated to have had a positive impact on health [ 20 ], which helps reduce the risk of subsequent suicide attempts. By contrast, cognitive decline is more likely to occur 2 years later after natural disasters [ 51 , 52 ], resulting from the new onset of depression and disruption of social contacts (e.g. loss of interactions with neighbours) [ 51 ].
Our findings align with previous studies highlighting the vulnerability of cancer patients to disruptions in healthcare services following natural disasters. While limited evidence suggests an increased risk of disease exacerbation among cancer patients post-disaster, our primary concern is the potential for delays in receiving essential cancer care [ 14 , 18 ]. Natural disasters like floods can severely disrupt healthcare systems, leading to damage to oncology centres, loss of medical records, pharmaceutical shortages, displacement of healthcare workers, and disruptions in pathology specimen handling, all of which can compromise cancer patient care [ 13 , 53 , 54 , 55 , 56 ]. The relocation of cancer patients to temporary shelters can be particularly challenging and distressing, especially for those with clinical instability [ 53 ]. Additionally, initial recovery efforts following natural disasters often prioritize immediate needs such as providing shelter, food, water, and addressing injuries from environmental hazards, infectious diseases, or other acute conditions [ 57 ]. This prioritization of immediate needs may inadvertently overlook the continuity of care required for non-acute medical issues like cancer. Given the individualized and continuous nature of cancer treatment, neoplasms are particularly susceptible to the disruptions caused by natural disasters. Our study demonstrates the association between floods and elevated mortality risks among cancer patients, reinforcing the urgent need to prioritize the needs of cancer patients before, during, and after disasters [ 13 , 58 ].
Profiles of vulnerable populations to flood-related mortality varied across causes of death. Of all factors considered, socio-economic status, which is determined by individual levels of education and income, has been identified as a significant modifier of flood-related mortality impacts. Individuals with higher socioeconomic status tend to have an increased risk of flood-related mortality from chronic diseases (e.g. cardiovascular diseases, respiratory diseases, and neurodegenerative diseases) but decreased risk of flood-related mortality from suicide. Although there is very limited evidence that can elucidate this finding, some insights can be gathered from the following studies. It is reported that people in high socioeconomic groups are more likely to be affected by work-life conflict-induced mental illness due to their higher occupational aspirations but a greater discrepancy between aspirations and reality [ 59 , 60 ]. Flooding exposure may further amplify the disparity between an individual’s aspirations and their actual circumstances, resulting in a negative impact on their mental health. Long-lasting psychological illness has been associated with worse chronic medical conditions [ 61 ].
In our study, we observed that participants with higher BMI and lower physical activity levels exhibited a significantly higher risk of flood-related mortality from suicide, but a comparatively lower risk of all-cause mortality. These associations can be attributed to different factors. On the one hand, individuals in low socio-economic groups, those engaged in minimal physical activity, and non-moderate alcohol drinkers, are at higher risk of developing suicide ideation in a short time following psychological trauma associated with flooding exposure [ 49 , 62 ]. On the other hand, individuals with higher levels of physical activity may be prone to engage in risk-taking behaviours during flooding events, potentially leading to increased mortality rates [ 63 ]. These behaviours could involve actions such as entering floodwaters to cross a river or stream, safeguarding property and families (e.g. through activities like sandbagging homes and clearing drains), and participating in rescuing operations [ 63 ]. Surprisingly, current smokers demonstrated a decreased risk of flood-related mortality from all-cause deaths and neoplasms. We acknowledge that residual confounding, raising from unmeasured factors at follow-up, might contribute to these associations. Nevertheless, it is important to note that our study represents the first report of a higher mortality risk after long-term exposure to floods, highlighting the need for further investigations to validate this finding and explore potential underlying reasons.
Based on our research, flooding exposure is responsible for advancing a substantial number of deaths, with the impact persisting for up to 6 years. Our findings suggest that preventive interventions should be implemented peri- and post-flooding periods to reduce avoidable deaths due to flooding exposure. Following a flooding event, there is an increased risk of suicide within the first year. Therefore, timely provision of coping support and stress management is crucial to avert psychological illness, particularly among individuals in low socio-economic groups, those engaged in less physical activity, and non-moderate alcohol drinkers. In long-term rehabilitation, more resources should be allocated towards addressing the chronic medical conditions of populations that have been exposed to flooding, especially neurological well-being. It is also crucial to pay attention to the high-income population, although further research is needed to elucidate the underlying mechanisms behind their greater mortality risks associated with flooding exposure.
The limitations merit consideration. Our participants were residents in the UK who were more likely to live in less socioeconomically deprived areas, therefore, our results may not be generalizable to a whole population, especially people in low- and middle-income countries. Like most of cohort studies, covariates were collected at enrolment in the biobank. Due to the limited information on behavioural changes after the baseline examinations, we are unable to exclude the effect of behavioural changes on the risk estimates. However, most of the covariates (e.g. socio-economic status) were considered as effect modifiers rather than confounders, therefore, any changes in these factors should not have a substantial impact on our estimates. While the flood index accounts for cumulative exposure, it does not yet capture the potential differential impacts of distinct flood phases (warning, event, post-event) on mortality. Further research with a short-term design would be helpful to investigate the impacts of distinct flood phases. The destructive power of floods can differ based on factors like terrain, altitude, water management, drainage, urbanization, and building design. Therefore, a single severity label for an entire flood event may not fully capture the nuances of varying local experiences. Further research is needed to refine our exposure assessment as more detailed data becomes available. Lastly, it is likely for people to move after flooding exposure, with or without moving back into their homes. We assumed that participants did not move, which may have underestimated the effect of floods if an individual moved from an area with a high risk of flooding to an area with a lower risk of flooding. However, exposure to flooding can still have a long-term impact on them due to potential property damage and financial loss, even if people relocate to areas with a low risk of flooding in the aftermath of the event.
In conclusion, this study provides robust epidemiological evidence for associations of long-term exposure to flooding with increased risk of mortality. The health consequences of flooding exposure can vary across different periods after the event. These findings contribute to a better understanding of the long-term impacts of flooding exposure and can help improve public health practices to reduce the disease burden associated with floods.
Availability of data and materials
Data used in this study are available through registration on the UK Biobank.
Abbreviations
Akaike Information Criterion
Bayesian Information Criterion
Body mass index
Confidence interval
Coronavirus disease 2019
Dartmouth Flood Observatory
European Centre for Medium-Range Weather Forecasts Reanalysis v5
International Classification of Diseases, edition 10
International Physical Activity Questionnaire-Short Form
Metabolic equivalent minutes per week
Post-traumatic stress disorder
Standard deviation
Townsend deprivation index
Lee J, Perera D, Glickman T, Taing LN. Water-related disasters and their health impacts: a global review. Prog Disaster Sci. 2020;8:100123.
Article Google Scholar
Wahlstrom M, Guha-Sapir D. The human cost of weather-related disasters 1995–2015. Geneva: UNISDR. 2015.
Wahlstrom M, Guha-Sapir D. The human cost of natural disasters: a global perspective. Geneva: UNISDR. 2015.
Rubinato M, Nichols A, Peng Y, Zhang JM, Lashford C, Cai YP, et al. Urban and river flooding: Comparison of flood risk management approaches in the UK and China and an assessment of future knowledge needs. Water Sci Eng. 2019;12(4):274–83.
Pitt M. Learning Lessons From the 2007 Floods. An Independent Review by Sir Michael Pitt. Cabinet Office, London. 2008.
Pregnolato M, Ford A, Wilkinson SM, Dawson RJ. The impact of flooding on road transport: A depth-disruption function. Transp Res D Transp Environ. 2017;55:67–81.
Thorne C. Geographies of UK flooding in 2013/4. Geogr J. 2014;180(4):297–309.
Marsh T, Kirby C, Muchan K, Barker L, Henderson E, Hannaford J. The winter floods of 2015/2016 in the UK-a review: UK: NERC/Centre for Ecology and Hydrology; 2016.
Wallemacq P, Below R, McClean D. Economic losses, poverty and disasters: 1998-2017: Geneva: United Nations Office for Disaster Risk Reduction; 2018.
Sayers P, Horritt M, Carr S, Kay A, Mauz J, Lamb R, et al. Third UK Climate Change Risk Assessment (CCRA3): Future flood risk. UK: Research undertaken by Sayers and Partners for the Committee on Climate Change; 2020.
Jonkman SN, Kelman I. An analysis of the causes and circumstances of flood disaster deaths. Disasters. 2005;29(1):75–97.
Article PubMed Google Scholar
Boyce R, Reyes R, Matte M, Ntaro M, Mulogo E, Metlay JP, et al. Severe Flooding and Malaria Transmission in the Western Ugandan Highlands: Implications for Disease Control in an Era of Global Climate Change. J Infect Dis. 2016;214(9):1403–10.
Article PubMed PubMed Central Google Scholar
Man RX, Lack DA, Wyatt CE, Murray V. The effect of natural disasters on cancer care: a systematic review. Lancet Oncol. 2018;19(9):e482–99.
Ryan B, Franklin RC, Burkle FM Jr, Aitken P, Smith E, Watt K, et al. Identifying and Describing the Impact of Cyclone, Storm and Flood Related Disasters on Treatment Management, Care and Exacerbations of Non-communicable Diseases and the Implications for Public Health. PLoS Curr. 2015;7:21–50.
Google Scholar
McKinney N, Houser C, Meyer-Arendt K. Direct and indirect mortality in Florida during the 2004 hurricane season. Int J Biometeorol. 2011;55(4):533–46.
Rath B, Young EA, Harris A, Perrin K, Bronfin DR, Ratarh R, et al. Adverse Respiratory Symptoms and Environmental Exposures Among Children and Adolescents Following Hurricane Katrina. Public Health Rep. 2011;126(6):853–60.
Buajaroen H. Management of health care services for flood victims: The case of the shelter at Nakhon Pathom Rajabhat University Central Thailand. Australas Emerg Nurs. 2013;16(3):116–22.
Loehn B, Pou AM, Nuss DW, Tenney J, McWhorter A, DiLeo M, et al. Factors affecting access to head and neck cancer care after a natural disaster: a post-Hurricane Katrina survey. Head Neck. 2011;33(1):37–44.
Alderman K, Turner LR, Tong S. Assessment of the health impacts of the 2011 summer floods in Brisbane. Disaster Med Public Health Prep. 2013;7(4):380–6.
Zhong S, Yang L, Toloo S, Wang Z, Tong S, Sun X, et al. The long-term physical and psychological health impacts of flooding: A systematic mapping. Sci Total Environ. 2018;626:165–94.
Article CAS PubMed Google Scholar
Trief PM, Ouimette P, Wade M, Shanahan P, Weinstock RS. Post-traumatic stress disorder and diabetes: co-morbidity and outcomes in a male veterans sample. J Behav Med. 2006;29(5):411–8.
Sharpe I, Davison CM. Climate change, climate-related disasters and mental disorder in low- and middle-income countries: a scoping review. BMJ Open. 2021;11(10):e051908.
Carozza DA, Boudreault M. A global flood risk modeling framework built with climate models and machine learning. J Adv Model Earth Syst. 2021;13(4):e2020MS002221.
Yang Z, Huang W, McKenzie JE, Xu R, Yu P, Ye T, et al. Mortality risks associated with floods in 761 communities worldwide: time series study. BMJ. 2023;383:e075081.
Steenland K, Seals R, Klein M, Jinot J, Kahn HD. Risk estimation with epidemiologic data when response attenuates at high-exposure levels. Environ Health Perspect. 2011;119(6):831–7.
White E, Hunt JR, Casso D. Exposure measurement in cohort studies: the challenges of prospective data collection. Epidemiol Rev. 1998;20(1):43–56.
Ganna A, Ingelsson E. 5 year mortality predictors in 498,103 UK Biobank participants: a prospective population-based study. Lancet. 2015;386(9993):533–40.
Wang M, Zhou T, Song Q, Ma H, Hu Y, Heianza Y, et al. Ambient air pollution, healthy diet and vegetable intakes, and mortality: a prospective UK Biobank study. Int J Epidemiol. 2022;51(4):1243–53.
Wang M, Zhou T, Song Y, Li X, Ma H, Hu Y, et al. Joint exposure to various ambient air pollutants and incident heart failure: a prospective analysis in UK Biobank. European heart journal. 2021;42(16):1582–91.
Article CAS PubMed PubMed Central Google Scholar
IPAQ Research Committee. Guidelines for data processing and analysis of the International Physical Activity Questionnaire (IPAQ)-short and long forms. https://www.physio-pedia.com/images/c/c7/Quidelines_for_interpreting_the_IPAQ.pdf (accessed January 20, 2024).
Zhang YB, Chen C, Pan XF, Guo J, Li Y, Franco OH, et al. Associations of healthy lifestyle and socioeconomic status with mortality and incident cardiovascular disease: two prospective cohort studies. BMJ. 2021;373:n604.
Chen H, Cao Y, Ma Y, Xu W, Zong G, Yuan C. Age- and sex-specific modifiable risk factor profiles of dementia: evidence from the UK Biobank. Eur J Epidemiol. 2023;38(1):83–93.
Jung CR, Chung WT, Chen WT, Lee RY, Hwang BF. Long-term exposure to traffic-related air pollution and systemic lupus erythematosus in Taiwan: A cohort study. Sci Total Environ. 2019;668:342–9.
Gasparrini A. Modeling exposure-lag-response associations with distributed lag non-linear models. Stat Med. 2014;33(5):881–99.
Li Y-Z, Huang S-H, Shi S, Chen W-X, Wei Y-F, Zou B-J, et al. Association of long-term particulate matter exposure with all-cause mortality among patients with ovarian cancer: A prospective cohort. Sci Total Environ. 2023;884:163748.
Kriit HK, Andersson EM, Carlsen HK, Andersson N, Ljungman PL, Pershagen G, et al. Using distributed lag non-linear models to estimate exposure lag-response associations between long-term air pollution exposure and incidence of cardiovascular disease. Int J Env Res Pub He. 2022;19(5):2630.
Article CAS Google Scholar
Rubin DB. Multiple imputation for nonresponse in surveys: Canada: Wiley; 2004.
Hashizume M, Wagatsuma Y, Faruque ASG, Hayashi T, Hunter PR, Armstrong B, et al. Factors determining vulnerability to diarrhoea during and after severe floods in Bangladesh. J Water Health. 2008;6(3):323–32.
Saulnier DD, Hanson C, Ir P, Alvesson HM, von Schreeb J. The Effect of Seasonal Floods on Health: Analysis of Six Years of National Health Data and Flood Maps. Int J Env Res Pub He. 2018;15(4):665–77.
Liu ZD, Lao JH, Zhang Y, Liu YY, Zhang J, Wang H, et al. Association between floods and typhoid fever in Yongzhou, China: Effects and vulnerable groups. Environ Res. 2018;167:718–24.
Rodriguez-Llanes JM, Ranjan-Dash S, Mukhopadhyay A, Guha-Sapir D. Flood-Exposure Is Associated with Higher Prevalence of Child Undernutrition in Rural Eastern India. Int J Env Res Pub He. 2016;13(2):210.
Milojevic A, Armstrong B, Kovats S, Butler B, Hayes E, Leonardi G, et al. Long-term effects of flooding on mortality in England and Wales, 1994–2005: controlled interrupted time-series analysis. Environ Health. 2011;10(1):11–9.
Milojevic A, Armstrong B, Hashizume M, McAllister K, Faruque A, Yunus M, et al. Health Effects of Flooding in Rural Bangladesh. Epidemiology. 2012;23(1):107–15.
Ahern M, Kovats RS, Wilkinson P, Few R, Matthies F. Global health impacts of floods: Epidemiologic evidence. Epidemiol Rev. 2005;27:36–46.
Tomio J, Sato H, Mizumura H. Interruption of Medication among Outpatients with Chronic Conditions after a Flood. Prehospital Disaster. 2010;25(1):42–50.
Turner LR, Alderman K, Huang CR, Tong SL. Impact of the 2011 Queensland floods on the use of tobacco, alcohol and medication. Aust Nz J Publ Heal. 2013;37(4):396.
Orri M, Seguin JR, Castellanos-Ryan N, Tremblay RE, Cote SM, Turecki G, et al. A genetically informed study on the association of cannabis, alcohol, and tobacco smoking with suicide attempt. Mol Psychiatr. 2021;26(9):5061–70.
Berlin I, Hakes JK, Hu MC, Covey LS. Tobacco Use and Suicide Attempt: Longitudinal Analysis with Retrospective Reports. Plos One. 2015;10(4):e0122607.
Darvishi N, Farhadi M, Haghtalab T, Poorolajal J. Alcohol-Related Risk of Suicidal Ideation, Suicide Attempt, and Completed Suicide: A Meta-Analysis. Plos One. 2015;10(5):e0126870.
Gobbi G, Atkin T, Zytynski T. Association of Cannabis Use in Adolescence and Risk of Depression, Anxiety, and Suicidality in Young Adulthood: A Systematic Review and Meta-analysis. Jama Psychiat. 2019;76(4):426–34.
Hikichi H, Aida J, Kondo K, Tsuboya T, Matsuyama Y, Subramanian SV, et al. Increased risk of dementia in the aftermath of the 2011 Great East Japan Earthquake and Tsunami. P Natl Acad Sci USA. 2016;113(45):E6911–8.
Akanuma K, Nakamura K, Meguro K, Chiba M, Gutierrez Ubeda SR, Kumai K, et al. Disturbed social recognition and impaired risk judgement in older residents with mild cognitive impairment after the Great East Japan Earthquake of 2011: the Tome Project. Psychogeriatrics. 2016;16(6):349–54.
Morikawa N, Yanagisawa S, Iwasashi H, Tabata T, Abe T, Nakamura R, et al. Cancer patients in the hospital damaged by the Japan earthquake and tsunami. J Clin Oncol. 2012;30(15):e19508-e.
Cancer Ullman K, Disasters Care During Natural. J Natl Cancer I. 2011;103(24):1819–20.
Arrieta MI, Foreman RD, Crook ED, Icenogle ML. Providing Continuity of Care for Chronic Diseases in the Aftermath of Katrina: From Field Experience to Policy Recommendations. Disaster Med Public. 2009;3(3):174–82.
Rosenthal E. How the Cancer Community Fared During Hurricane Sandy's Mid-Atlantic Sweep. Oncology Times. 2012;34(23):8-10.
Prasad AS, Francescutti LH. Natural disasters. International encyclopedia of public health. 2017;5:215-22.
Aitsi-Selmi A, Murray V. Protecting the Health and Well-being of Populations from Disasters: Health and Health Care in The Sendai Framework for Disaster Risk Reduction 2015–2030. Prehospital Disaster. 2016;31(1):74–8.
Cochran DB, Wang EW, Stevenson SJ, Johnson LE, Crews C. Adolescent Occupational Aspirations: Test of Gottfredson’s Theory of Circumscription and Compromise. Career Dev Q. 2011;59(5):412–27.
Kim YM, Cho SI. Socioeconomic status, work-life conflict, and mental health. Am J Ind Med. 2020;63(8):703–12.
Patten SB, Williams JVA, Lavorato DH, Modgill G, Jette N, Eliasziw M. Major depression as a risk factor for chronic disease incidence: longitudinal analyses in a general population cohort. Gen Hosp Psychiat. 2008;30(5):407–13.
Vancampfort D, Hallgren M, Firth J, Rosenbaum S, Schuch FB, Mugisha J, et al. Physical activity and suicidal ideation: A systematic review and meta-analysis. J Affect Disorders. 2018;225:438–48.
Hamilton K, Demant D, Peden AE, Hagger MS. A systematic review of human behaviour in and around floodwater. Int J Disaster Risk Reduction. 2020;47:101561.
Download references
Acknowledgements
We gratefully thank all the participants in the UK Biobank, and everyone involved in planning and conducting the study.
Australian Research Council grant DP210102076
Australian National Health and Medical Research Council grant GNT2000581)
China Scholarship Council grant 202006010044 (YW)
China Scholarship Council grant 202006010043 (BW)
China Scholarship Council grant 201906210065 (PY)
National Health and Medical Research Council grant GNT2009866 (SL)
National Health and. Medical Research Council grant GNT1163693 (YG)
National Health and Medical Research Council grant GNT2008813 (YG)
Author information
Hong Liu, Shanshan Li, and Yuming Guo are co-senior authors.
Authors and Affiliations
School of Public Health and Preventive Medicine, Monash University, Level 2, 553 St Kilda Road, Melbourne, VIC, 3004, Australia
Yao Wu, Danijela Gasevic, Bo Wen, Zhengyu Yang & Pei Yu
Department of Dermatology, Xiangya Hospital, Central South University, Changsha, 410008, Hunan, China
Guowei Zhou, Yan Zhang, Hong Liu, Shanshan Li & Yuming Guo
Department of Biochemistry and Molecular Biology, Monash Biomedicine Discovery Institute, Monash University, Melbourne, VIC, 3800, Australia
Jiangning Song
You can also search for this author in PubMed Google Scholar
Contributions
Conceptualization: YG, JS, HL, SL, YW. Methodology: YW, DG. Data collection: BW, ZY, GZ, YZ, ZY
Visualization: YW, BW. Supervision: YG, HL, SL. Writing—original draft: YW. Writing—review and editing: DG, BW, ZY, PY. All authors read and approved the final manuscript.
Authors’ Twitter handles
Yuming Guo: @YumingGuo007
Yao Wu: @Yaoyaowu13
Corresponding author
Correspondence to Yuming Guo .
Ethics declarations
Ethics approval and consent to participate.
UK Biobank has ethical approval from the North West Multi-Centre Research Ethics Committee (reference 16/NW/0274).
Consent for publication
Consent for publication was obtained from all included participants.
Competing interests
The authors declare that they have no competing interests.
Additional information
Publisher’s note.
Springer Nature remains neutral with regard to jurisdictional claims in published maps and institutional affiliations.
Supplementary Information
Additional file 1:.
Table S1. Definitions of different severities of flood events. Table S2. The Akaike Information Criterion (AIC) and the Bayesian Information Criterion (BIC) of nonlinear and linear models. Table S3. Baseline characteristics of cases and matched controls enrolled in UK Biobank, including missing values. Table S4. Cumulative odds ratios of cause-specific mortality associated with per unit increase in flood index over lag years 0–5. Table S5. Cumulative odds ratios of all-cause mortality associated with per unit increase in monthly flood index over lag month 0–12. Figure S1. Nonlinear curves of the associations between flood index and all-cause and cause-specific mortality. Figure S2. Cumulative flood index of cases and controls during the six years before the date of death or the end of the follow-up. Figure S3. Cumulative odds ratios of all-cause and cause-specific mortality associated with per unit increase in flood index over lag years 0–5 using complete data after multiple imputation. Figure S4. Cumulative odds ratios of all-cause and cause-specific mortality associated with per unit increase in flood index over lag years 0–5 using different degrees of freedom for lag-response association of flood index. Figure S5. Cumulative odds ratios of all-cause and cause-specific mortality associated with per unit increase in flood index over lag years 0–5 using different degrees of freedom for mean temperature. Figure S6. Cumulative odds ratios of all-cause and cause-specific mortality associated with per unit increase in flood index over lag years 0–5 using different degrees of freedom for relative humidity. Figure S7. Cumulative odds ratios of all-cause and cause-specific mortality associated with per unit increase in flood index over lag years 0–5 after excluding deaths after 2020. Figure S8. Cumulative odds ratios of all-cause and cause-specific mortality associated with per unit increase in flood index over lag years 0–5 using different matching ratios.
Rights and permissions
Open Access This article is licensed under a Creative Commons Attribution 4.0 International License, which permits use, sharing, adaptation, distribution and reproduction in any medium or format, as long as you give appropriate credit to the original author(s) and the source, provide a link to the Creative Commons licence, and indicate if changes were made. The images or other third party material in this article are included in the article's Creative Commons licence, unless indicated otherwise in a credit line to the material. If material is not included in the article's Creative Commons licence and your intended use is not permitted by statutory regulation or exceeds the permitted use, you will need to obtain permission directly from the copyright holder. To view a copy of this licence, visit http://creativecommons.org/licenses/by/4.0/ . The Creative Commons Public Domain Dedication waiver ( http://creativecommons.org/publicdomain/zero/1.0/ ) applies to the data made available in this article, unless otherwise stated in a credit line to the data.
Reprints and permissions
About this article
Cite this article.
Wu, Y., Gasevic, D., Wen, B. et al. Floods and cause-specific mortality in the UK: a nested case-control study. BMC Med 22 , 188 (2024). https://doi.org/10.1186/s12916-024-03412-0
Download citation
Received : 27 November 2023
Accepted : 29 April 2024
Published : 07 May 2024
DOI : https://doi.org/10.1186/s12916-024-03412-0
Share this article
Anyone you share the following link with will be able to read this content:
Sorry, a shareable link is not currently available for this article.
Provided by the Springer Nature SharedIt content-sharing initiative
- Natural disaster
BMC Medicine
ISSN: 1741-7015
- Submission enquiries: [email protected]
- General enquiries: [email protected]
Practical flood risk reduction strategies in South Sudan
Affiliations.
- 1 Emergency & Disaster Management, Military Intelligence, Emergency and Disaster Management, Master's Program, School of Continuing Studies, Georgetown University, Washington, DC.
- 2 Adjunct Faculty (Ethics, Research, GIS), Emergency and Disaster Management, Master's Program, School of Continuing Studies, Georgetown University, Washington, DC. ORCID: https://orcid.org/0000-0003-0531-6314.
- 3 Professor of the Practice and Faculty Director, School of Continuing Studies (SCS), Emergency and Disaster Management, Master's Program, School of Continuing Studies, Georgetown University, Washington, DC. ORCID: https://orcid.org/0000-0003-1270-5078.
- PMID: 36825636
- DOI: 10.5055/jem.0669
More extreme weather patterns caused by climate change are leading to more intense and frequent flooding in some of the world's most vulnerable locations. The Republic of South Sudan faces obstacles mitigating rivers and urban floods caused by heavy rainfall in many of its regions. Through a focused review of the related literature, this study will first analyze the effectiveness and failures of the South Sudanese government's attempts to mitigate flood risks, save lives, and prevent property damage. Study data are derived from various sources including peer-reviewed research, government and nongovernment documents, and the news media. Next, using similar qualitative review methods, a parallel case study from Bangladesh will be used to glean comparative findings and help formulate flood risk reduction recommendations for South Sudan. Findings include that government efforts are highly reactive and lack adequate resources to address flood mitigation. Analysis also indicates that locally driven structural and nonstructural disaster risk reduction efforts are critical to reducing vulnerability to this ongoing and intensifying threat. The study implications and recommendations advance the existing literature on this topic and are relevant to any global region displaying vulnerability opposite the complex threat of repeated intense flooding.
Publication types
- Climate Change
- South Sudan
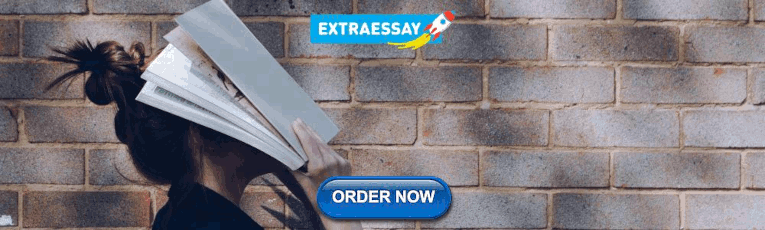
IMAGES
VIDEO
COMMENTS
Water is a limited resource but essential to sustaining life and supporting economic development. Only 2.5% of all the water on Earth is freshwater and can be used to meet basic human needs such as a clean supply of water for drinking, cooking, and bathing. Water scarcity is the result of an imbalance between supply and demand. Efficient water resource management is definitely of interest to ...
Identifying the areas at risk of river flooding, urban flooding, and coastal flooding is a complicated process, as the causes of these events differ. Although it is known that flood risk increases with ... assessed the effectiveness of NBS projects and demonstrated that NBS has positive effects on flood risk reduction and climate change ...
However, the number of fatalities and people affected has decreased since the mid-1990s because of risk reduction measures, such as improved risk awareness and structural flood defences.
the w orst flooding in 30 years that ba ttered W est Africa from July 200 7 caused more. than 21 0 death and aff ected more than 78 5,000 people [ 12]. The aftermaths of flood disasters in Ghana ...
1 INTRODUCTION. Flood risk management (FRM) aims to balance the negative consequences of floods with societal goals such as development and environmental preservation (Klijn et al., 2009; WMO & GWP, 2007).This balance implies challenging decisions, not only because they arise from conflicts among outcomes that people value, but because they are always related to the future and are often based ...
1. The flood risk reduction and management is very efficient. 2. There is a significant difference between the subdomains of the. flood risk reduction and management. 1. The practices in managing ...
Modern-day flood management has evolved into a variety of flood management alternatives. The selection of appropriate flood measures is crucial under a variety of flood management practices, approaches, and assessment criteria. Many leading countries appraise the significance of risk-based flood management, but the fixed return period is still the de facto standard of flood management practices.
Risk management has reduced vulnerability to floods and droughts globally1,2, yet their impacts are still increasing3. An improved understanding of the causes of changing impacts is therefore ...
Urban areas in Ghana have experienced worsening floods in terms of scale and number of affected people. In many instances, attribution has been made to one dominant factor as the cause of the rising flood incidence. The nature of the problem however suggests a complex interplay of factors at work but which have received limited research attention. Drawing on climatic data, household and key ...
Most research on hydrological risks focuses either on flood risk or drought risk, whilst floods and droughts are two extremes of the same hydrological cycle. To better design disaster risk reduction (DRR) measures and strategies, it is important to consider interactions between these closely linked phenomena. We show examples of: (a) how flood ...
However, not all disasters can be prevented. Effective adaptation to rising flood risk requires a diversified approach of interventions, which may include structural flood protection measures, early warning systems, risk-informed land planning, nature-based solutions, social protection and risk financing instruments 13.
This essay focuses on the role of local communities during the recovery stage from floods. By drawing on a case study of rural communities in Laos, the essay highlights the potential benefits of equipping local communities with long-term practices that will assist them in recovery efforts. The essay concludes with a discussion of the lessons and insights gained from this case and that they ...
Drains also increase water flow into surrounding rivers, increasing the flood risk. Agricultural practices can influence how water moves. For instance, fields without crops, especially during winter when soils are wet, can quickly channel water. Also, ploughing down slopes can form small channels that accelerate water flow directly to rivers.
3.1 Probabilistic Flood Risk Assessment, Climate Change, and Resilience: The Case of La Mojana, Colombia. La Mojana, located in the northwest of Colombia, is an alluvial delta of more than one million hectares where the Magdalena River, the Cauca River, and the San Jorge River converge. It is an extremely flat land with multiple water channels ...
In the end, 80 papers were selected in the final round for this systematic review. Figures 2 and 3 depict the results of the searches on flooding in Nigeria and Ghana, respectively. Data for 2021 in the charts cover only the first few months of the year. ... Understanding the Causes for Flood Risk Reduction in Kumasi, Ghana." GeoJournal ...
Disaster Risk reduction (DRR) aims to reduce the damage caused by natural hazards like earthquakes, floods, droughts and cyclones, through an ethic of prevention. Disasters often follow natural hazards. The Hyogo Framework is a global blueprint for disaster risk reduction efforts during the next decade. Its goal is to substantially reduce ...
Flooding is a natural disaster that coexists with human beings and causes severe loss of life and property worldwide. Although numerous studies for flood susceptibility modelling have been introduced, a notable gap has been the overlooked or reduced consideration of the uncertainty in the accuracy of the produced maps. Challenges such as limited data, uncertainty due to confidence bounds, and ...
Natural hazards are one of the destructive phenomena that pose a significant hazard to humans, property, and the economy, among others. One of the most recurring natural hazards is flooding, which is caused by typhoons, monsoons, and heavy rainfall and has been one of the main concerns of the Philippines in recent years. The study's results will provide information on the factors affecting ...
Vietnam is exposed to different types of floods that cause severe economic losses, damage to infrastructure, and loss of life. Reliable information on the drivers, patterns and dynamics of flood risk is crucial for the identification, prioritization and planning of risk reduction and adaptation measures.
Semantic Scholar extracted view of "Theoretical Framework Development for Flood Vulnerable Communities Using Geographic Information System for Disaster Risk Reduction: Mediating Role of Flood Risk Perception" by S. K. Abid et al.
The climatic conditions of the earth have changed significantly over the centuries. This can be noticed from the geological evidence of changes in sea levels and ice ages. The patterns of human activity over the centuries also give good evidence of climate change. We will write a custom essay on your topic. 809 writers online.
Decision-support tools like multi-criteria analysis can address by enabling stakeholder collaboration. This research aims to asses the utility of EBA strategies for flood risk reduction and offering co-benefits in diverse coastal European regions using multi-criteria analysis.
Floods pose a significant threat to road networks globally, disrupting transportation, isolating communities, and causing economic losses. This study proposes a four-stage methodology (avoidance, endurance, recovery, and adaptability) to enhance the resilience of road networks. We combine analysis of constructed assets and asset system performance with multiple disaster scenarios (Reactive ...
500+ words Essay on Flood. Flood is one of the most dangerous natural disasters. It happens when excessive water is collected in any area. It usually happens due to heavy rainfall. India is highly prone to flood. There are many regions in the country that face this natural disaster because of the overflowing of rivers.
500 Words Essay on Importance Of Disaster Risk Reduction Understanding Disaster Risk Reduction. Disaster Risk Reduction, or DRR, is a way of making sure that when natural events like earthquakes or floods happen, they cause as little harm as possible to people, their homes, and their way of life.
Floods are the most frequent type of weather-related disaster, accounting for about 47% of all weather-related disasters from 1995 to 2015 [1, 2].Between 1995 and 2015, more than 2.3 billion people were affected by flood disasters, with over 157 thousand people dying directly as a result of floods [].In recent years, many intense urban flooding events have been recorded in the UK, resulting in ...
Floods are caused by many things. Many times it rains too much, other times a dam breaks; however, the effects of floods devastating. Floods can cause environmental losses as well and economical losses, land is washed away, homes are ruined, and people sometimes even die. This essay is about the causes and effects that flood have on our world.
The Republic of South Sudan faces obstacles mitigating rivers and urban floods caused by heavy rainfall in many of its regions. Through a focused review of the related literature, this study will first analyze the effectiveness and failures of the South Sudanese government's attempts to mitigate flood risks, save lives, and prevent property ...
Quantifying flood risks through a cascade of hydraulic-cum-hydrodynamic modelling is data-intensive and computationally demanding- a major constraint for economically struggling and data-scarce low and middle-income nations. Under such circumstances, geomorphic flood descriptors (GFDs), that encompass the hidden characteristics of flood propensity may assist in developing a nuanced ...