We will keep fighting for all libraries - stand with us!
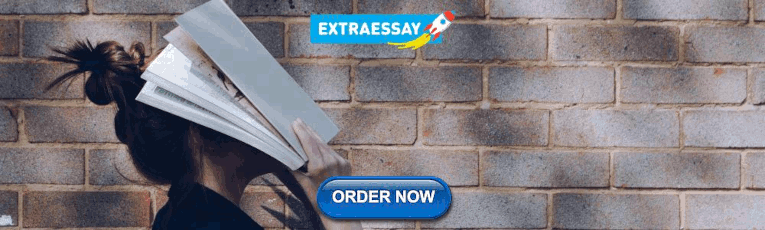
Internet Archive Audio
- This Just In
- Grateful Dead
- Old Time Radio
- 78 RPMs and Cylinder Recordings
- Audio Books & Poetry
- Computers, Technology and Science
- Music, Arts & Culture
- News & Public Affairs
- Spirituality & Religion
- Radio News Archive
- Flickr Commons
- Occupy Wall Street Flickr
- NASA Images
- Solar System Collection
- Ames Research Center
- All Software
- Old School Emulation
- MS-DOS Games
- Historical Software
- Classic PC Games
- Software Library
- Kodi Archive and Support File
- Vintage Software
- CD-ROM Software
- CD-ROM Software Library
- Software Sites
- Tucows Software Library
- Shareware CD-ROMs
- Software Capsules Compilation
- CD-ROM Images
- ZX Spectrum
- DOOM Level CD

- Smithsonian Libraries
- FEDLINK (US)
- Lincoln Collection
- American Libraries
- Canadian Libraries
- Universal Library
- Project Gutenberg
- Children's Library
- Biodiversity Heritage Library
- Books by Language
- Additional Collections
- Prelinger Archives
- Democracy Now!
- Occupy Wall Street
- TV NSA Clip Library
- Animation & Cartoons
- Arts & Music
- Computers & Technology
- Cultural & Academic Films
- Ephemeral Films
- Sports Videos
- Videogame Videos
- Youth Media
Search the history of over 866 billion web pages on the Internet.
Mobile Apps
- Wayback Machine (iOS)
- Wayback Machine (Android)
Browser Extensions
Archive-it subscription.
- Explore the Collections
- Build Collections
Save Page Now
Capture a web page as it appears now for use as a trusted citation in the future.
Please enter a valid web address
- Donate Donate icon An illustration of a heart shape
Introduction to engineering design : modelling, synthesis and problem solving strategies
Bookreader item preview, share or embed this item, flag this item for.
- Graphic Violence
- Explicit Sexual Content
- Hate Speech
- Misinformation/Disinformation
- Marketing/Phishing/Advertising
- Misleading/Inaccurate/Missing Metadata
Tight margin cut off text due to tight binding
![[WorldCat (this item)] [WorldCat (this item)]](https://archive.org/images/worldcat-small.png)
plus-circle Add Review comment Reviews
4 Favorites
DOWNLOAD OPTIONS
No suitable files to display here.
IN COLLECTIONS
Uploaded by station26.cebu on December 26, 2019
SIMILAR ITEMS (based on metadata)
We found a match
Your institution may have access to this item. Find your institution then sign in to continue.
Engineering Design Synthesis
Understanding, Approaches and Tools
- © 2002
- Amaresh Chakrabarti (Professor Associate) 0
Centre for Product Design and Manufacturing (CPDM), Indian Institute of Science, Bangalore Karnataka, India
You can also search for this editor in PubMed Google Scholar
- The first ever attempt to bring together a comprehensive array of some of the most influential pieces of research that together underpin today's understanding of what constitutes and influences design synthesis, and approaches as to how this important activity can be supported
- Broadens the approach to design from the traditional engineering point of view to include systematised research in the psychological and sociological effects of design
10k Accesses
104 Citations
This is a preview of subscription content, log in via an institution to check access.
Access this book
- Available as PDF
- Read on any device
- Instant download
- Own it forever
- Compact, lightweight edition
- Dispatched in 3 to 5 business days
- Free shipping worldwide - see info
- Durable hardcover edition
Tax calculation will be finalised at checkout
Other ways to access
Licence this eBook for your library
Institutional subscriptions
Table of contents (19 chapters)
Front matter, understanding, defining synthesis: on the senses and the logic of design synthesis.
- Norbert F. M. Roozenburg
Insight, design principles and systematic invention
- Michael J. French
Synthesis and theory of knowledge: general design theory as a theory of knowledge, and its implication to design
- Yoram Reich
Theory of technical systems and engineering design synthesis
- Vladimir Hubka, W. Ernst Eder
A knowledge operation model of synthesis
- Tetsuo Tomiyama, Masaharu Yoshioka, Akira Tsumaya
Two approaches to synthesis based on the domain theory
- Claus Thorp Hansen, Mogens Myrup Andreasen
Using the concept of functions to help synthesise solutions
- Gerhard Pahl, Ken Wallace
Design catalogues and their usage
- Karlheinz Roth
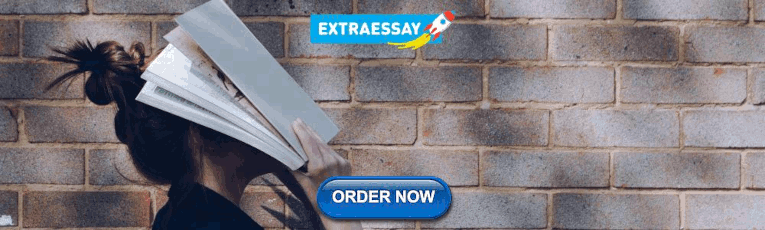
TRIZ, the Altshullerian approach to solving innovation problems
- Denis Cavallucci
Synthesis of schematic descriptions in mechanical design
- Karl T. Ulrich, Warren P. Seering
An approach to compositional synthesis of mechanical design concepts using computers
- Amaresh Chakrabarti, Patrick Langdon, Yieng-Chieh Liu, Thomas P. Bligh
Synthesis based on function-means trees: Schemebuilder
- Rob Bracewell
Design processes and context for the support of design synthesis
- Ralf-Stefan Lossack
Retrieval using configuration spaces
- Tamotsu Murakami
Creative design by analogy
Design patterns and creative design.
- Sambasiva R. Bhatta, Ashok K. Goel
- Theorie des erfinderischen Problemlösens
- Theory of Inventive Problem Solving
- computer-aided conceptual design
- conceptual design
- design process
- design theory
- engineering design
- product development
- product development methods
- product innovation
- programming
About this book
Editors and affiliations.
Amaresh Chakrabarti
Bibliographic Information
Book Title : Engineering Design Synthesis
Book Subtitle : Understanding, Approaches and Tools
Editors : Amaresh Chakrabarti
DOI : https://doi.org/10.1007/978-1-4471-3717-7
Publisher : Springer London
eBook Packages : Springer Book Archive
Copyright Information : Springer-Verlag London 2002
Hardcover ISBN : 978-1-85233-492-5 Published: 20 February 2002
Softcover ISBN : 978-1-84996-876-8 Published: 15 December 2010
eBook ISBN : 978-1-4471-3717-7 Published: 09 March 2013
Edition Number : 1
Number of Pages : XXX, 330
Topics : Engineering Design , Simulation and Modeling
- Publish with us
Policies and ethics
- Find a journal
- Track your research
(Stanford users can avoid this Captcha by logging in.)
- Send to text email RefWorks EndNote printer
Introduction to engineering design : modelling, synthesis and problem solving strategies
Available online.
- EBSCO Academic Comprehensive Collection
More options
- Find it at other libraries via WorldCat
- Contributors
Description
Creators/contributors, contents/summary.
- PART 1: MODELLING AND SYNTHESIS: Introducing Modelling and Synthesis for Structural Integrity; Design against failure; Design Synthesis of Generic Engineering Components; Design of Mechanical Connections; Review: Structural Integrity of Systems.
- PART 2: PROBLEM SOLVING STRATEGIES: AN ENGINEERING CULTURE: The Evolution of Design Problems; Economic and Environmental Issues: The 'Big Picture'
Bibliographic information
Browse related items.

- Stanford Home
- Maps & Directions
- Search Stanford
- Emergency Info
- Terms of Use
- Non-Discrimination
- Accessibility
© Stanford University , Stanford , California 94305 .
- Architecture and Design
- Asian and Pacific Studies
- Business and Economics
- Classical and Ancient Near Eastern Studies
- Computer Sciences
- Cultural Studies
- Engineering
- General Interest
- Geosciences
- Industrial Chemistry
- Islamic and Middle Eastern Studies
- Jewish Studies
- Library and Information Science, Book Studies
- Life Sciences
- Linguistics and Semiotics
- Literary Studies
- Materials Sciences
- Mathematics
- Social Sciences
- Sports and Recreation
- Theology and Religion
- Publish your article
- The role of authors
- Promoting your article
- Abstracting & indexing
- Publishing Ethics
- Why publish with De Gruyter
- How to publish with De Gruyter
- Our book series
- Our subject areas
- Your digital product at De Gruyter
- Contribute to our reference works
- Product information
- Tools & resources
- Product Information
- Promotional Materials
- Orders and Inquiries
- FAQ for Library Suppliers and Book Sellers
- Repository Policy
- Free access policy
- Open Access agreements
- Database portals
- For Authors
- Customer service
- People + Culture
- Journal Management
- How to join us
- Working at De Gruyter
- Mission & Vision
- De Gruyter Foundation
- De Gruyter Ebound
- Our Responsibility
- Partner publishers

Your purchase has been completed. Your documents are now available to view.
Solving Authentic Problems through Engineering Design
The importance of STEAM education in preparing students to deal with societal challenges is nowadays an international recommendation. We may find different perspectives concerning STEAM education and the integration of its disciplines. A possible pathway to achieve a balanced integration is through Engineering Design (ED), starting from problems of reality that enable the articulated mobilization of concepts from various disciplinary areas, promoting interdisciplinary where teamwork and context play a fundamental role. This article reports a study with primary preservice teachers (6–12 years old) that aims to analyze the learning displayed and the difficulties underlying the usage of ED in solving STEAM problems. The participants were 45 preservice teachers. We adopted a qualitative methodology, and data were collected through observation, documents, artifacts, and photos. The preliminary results show a very positive reaction of the participants to the experience of solving real context problems collaboratively and mobilizing mathematical and physical science concepts in an integrated way. We identified active engagement, persistence, and motivation in the creation of a model that fulfilled the requested conditions. Difficulties were evidenced in the identification of some concepts and the mobilization of adequate scientific language in the argumentation of decisions.
1 Introduction
The world is constantly changing and demanding new and complex challenges. Therefore, there is a growing emphasis on encouraging creative thinking in education, innovating pedagogies, and developing connections among subjects. In this scenario, there has been great emphasis on the integration that STEAM education can provide and how its disciplines should be articulated, which is still an open discussion (English & King, 2015 , 2019 ). One way for this integration could be using authentic problems solved through an Engineering Design (ED) process that involves students in active learning (e.g., Edwards, 2015 ; Edwards, Kemp, & Page, 2014 ; Vale & Barbosa, 2020 ). This means that students must be involved in meaningful learning through the implementation of individual and collaborative experiences that promote the development of internationally recommended skills like being creative, thinking critically, solving problems and making decisions, communicating, and collaborating – skills known as the 4Cs (NCTM, 2014 ; Schleicher, 2016 ; WEF, 2016 ).
As a result, the STEAM approach arises in the context of active learning, allowing connections to be created, where the use of challenging problems in authentic contexts that appeal to multiple approaches and/or solutions promotes the 4C’s skills, which are often lacking in more traditional classes. In this setting, engineering-based tasks’ solutions provide a rich source of meaningful situations that capitalize on and extend students’ learning, where ED serves as a rich source of attracting authentic contexts that draw on school mathematics, science, and technology. By integrating these tasks into the curricula, students have the opportunity to see the usefulness of what they learn in school about mathematics and science, when solving problems in the world outside of school.
Taking the previous ideas into consideration, in this article we report an ongoing study with primary preservice teachers (6–12 years old) in which we sought to understand and characterize the learning and reactions underlying the use of ED in solving authentic problems in a STEAM context. In particular, we are interested in the performance of these future teachers, along with a learning experience, based on solving an authentic problem, following the ED cycle, and identifying the main difficulties and the main contents mobilized in the process.
2 Theoretical Framework
The next two sections frame our study from a theoretical point of view. We briefly describe the need for active learning and an interdisciplinary approach through the STEAM perspective, focusing on the engineering approach.
2.1 Active Learning in Mathematics Classes
There are significant societal and economic changes and challenges that are being faced across the world as a result of the rapid level of technological innovation, a development that is altering the economic and social support of our lives. These changes have had a significant impact on the labor market and education. Students can no longer face this new world through instructions focused only on routine skills, ideas, and procedures. Much of the failure in school mathematics have their origin in the affective environment and the degree of challenge created in the classroom. The classroom practices under a traditional approach are yet grounded on memory and repetition, where mathematics is dealt with as a collection of isolated topics based on techniques and formulas to be memorized and practiced. This perspective can seriously compromise students’ initial expectations and motivations in relation to the curricular contents, their beliefs about mathematics as a subject, and their beliefs about themselves in relation to mathematics (e.g., Diego-Mantecón, Arcera, Blanco, & Lavicza, 2019 ; Diego-Mantecón, Ortiz-Laso, & Blanco, 2022 ; Hannula, 2001 ), so we have to propose an environment that reverses this situation. We need an exploratory classroom where we use an inquiry-based learning approach, a term that refers to classroom practices in which students understand, pose questions, explore, and discuss (Maass, Doorman, Jonker, & Wijers, 2019 ). This type of classroom is focused on learning; students learn by doing, understanding, analyzing, and discussing multiple approaches when solving a task. The role of the teacher is more demanding, and the instructional strategies and tasks selected are fundamental to achieving that initial goal. In this context, active learning arises, generally defined as an instructional method that involves students in the learning process (e.g., Edwards et al., 2014 ; Edwards, 2015 ; Prince, 2004 ). Active learning has its roots in socio-constructivist learning theory and advocates a classroom practice that engages students in activities such as talking, listening, reading, writing, discussing, and reflecting on content through problem-solving in small groups, simulations, or other activities. It requires the engagement of students in meaningful activities, through which they are constantly challenged to think about what they are doing. The focus is entirely on the students and the activity developed, as opposed to the more traditional approach in which they are limited to passively accessing the information transmitted by the teacher. For this, it is necessary that they solve meaningful tasks. The learning of mathematics must include diversified tasks that go beyond routine tasks, focusing particularly on problem-solving. It is sought that students internalize a set of strategies that allow them to expand their repertoire and become increasingly competent in mathematics “with” the approach to problem-solving (Barbosa & Vale, 2018 ; Liljedahl, 2016 ). Tasks with multiple solutions and involving authentic problems in real contexts should be privileged.
Active learning is grounded in an inquiry-based learning approach and includes the learners’ engagement through three dimensions: intellectual, social, and physical. The intellectual or cognitive dimension is related to problem-solving tasks in which students must explain and justify their reasoning. These tasks should also trigger social and physical activity to promote active learning. That is, intellectual engagement may not be enough, and learning needs a social component in which students confront ideas, allowing them to work collaboratively as a significant aspect of classrooms, where the role of communication and mathematical discussion stands out. This type of collaboration facilitates the sharing and development of mathematical meanings, and it is up to the teacher to foster a sense of community so that students feel safe and confident to take risks and express their ideas, either between peers or with the teacher (Barbosa & Vale, 2018 ; Edwards et al., 2014 ; Vale & Barbosa, 2021 ). The physical dimension is related to movement. Learning requires movement because an active body stimulates the brain, making students more engaged and attentive, improving comprehension and memorization, which contributes to a better performance (Hannaford, 2005 ). Students, especially the youngest, need to be physically active in the classroom, and this can be solved with the use of instructional active strategies such as hands-on projects, lab experiments, manipulatives, building models, math trails, or gallery walks, which also facilitate interactions. At the same time, these instructional strategies can serve to promote connections between contents, subjects, and representations (e.g., Barbosa & Vale, 2018 ; Hannaford, 2005 ; Vale & Barbosa, 2021 ).
2.2 From STEAM Education to ED
Another aspect that contributes to the failure and lack of motivation from students toward school and certain subjects is related to the approach of isolated themes/contents, without articulation, whether intra- or inter-subject. An idea or concept is better comprehended if one understands how it relates to or connects with other ideas or concepts that are already known (Hiebert et al., 1997 ); thus, interdisciplinarity and collaborative practices play a fundamental role in giving meaning to what students learn. Traditional classes have been changing their practices to more active ones, where the STEAM (Science, Technology, Engineering, Arts, and Mathematics) approach emerges in the context of active learning. STEAM education should involve not only teaching these core subjects and contents in isolation but also an interdisciplinary approach, building on content knowledge developed within and across these disciplines.
STEAM education may be guided by the adoption of different models: the approach of each area in isolation; STEAM as a discipline; STEAM approach through mathematics; STEAM approach through science; STEAM through ED. Knowing the schools’ reality and the organization of curricula, it is considered that the approach through a classic subject of the curriculum is easier to implement by teachers in either primary education or upper levels. Because mathematics and science are traditional subjects of the school curriculum, it is natural that teachers find it easier to use STEAM education with one of these subjects as a starting point rather than embarking on a STEAM program per se, as STEAM does not exist in the curricular matrix in many countries. An issue of great concern for many countries across Europe is the students’ poor achievement in math and sciences, particularly in problem-solving and inquiry tasks, which may be an obstacle to making certain career choices, directly related to those subjects, for instance, an engineering profession. These results can, in many cases, be related to the use of routine tasks, frequently deprived of meaning. In particular, in mathematics education, we have to be aware of this situation and adopt a mathematical practice that contemplates integration and interdisciplinarity (e.g., Sriraman & Freiman, 2009 ). We endorse the ideas of Martín-Páez, Aguilera, Perales-Palacios, and Vílchez-González ( 2019 ), who advocate for STEAM learning as the integration of conceptual, procedural, and attitudinal contents through a group of STEM skills for the application of ideas or the solving of interdisciplinary problems in real contexts. In this perspective, we advocate STEAM education as a teaching approach in which students build and demonstrate understanding and/or knowledge through engagement in an ED process in which science, technology, engineering, arts, and mathematics work together.
We consider that ED is an approach that promotes a bridge between math and science concepts, as well as arts, starting from problems from the real world that foster creativity, collaboration, decision-making, critical thinking, communication, and reasoning and promoting a natural STEAM integration. There are several frameworks for ED, but it is consensual that it is a cyclic process, composed of steps, leading to the achievement of a certain objective. The adapted ED cycle ( Figure 1 ) from several authors (Cunningham & Hester, 2007 ; Design Squad Global, 2019 ; English & King, 2015 ) is thus composed of seven steps, namely: problem (define the problem/identify the constraints); imagine (brainstorm ideas/look for possible solutions/choose the best one); design (plan the solution/draw a sketch); (re)build (follow the plan and create and construct the idea); (re)test and evaluate (test and evaluate the idea/the prototype); redesign (discuss what works/what does not work/improve/modify your design to make it better/test it once more); and solution (share and communicate the solution/results/obtained product).

The ED cycle (adapted from Cunningham & Hester, 2007 ).
An integrated STEAM instruction grounded in ED uses the practices of engineering as the context and/or an intentional component of the content to be learned, acting as an “integrator” that brings together different parts in a way that requires them to work together as a whole (Bryan, Moore, Johnson, & Roehrig, 2015 ). The implementation of ED requires the use of real-life problems, which many authors call authentic tasks. Authentic tasks employ realistic data, providing rich information about the situation described, and can be solved through different ways and could have different kinds of solutions. They always ask for the application of specific knowledge appropriate to the situation and specific skills in accordance with real-life challenges (Kramarski & Mevarech, 2014 ). Teachers are the key for this change to occur, so we believe that they need to develop their abilities, through training, to make a difference in the future with their own students. Furthermore, throughout ED, students must manage risk and uncertainty while solving the given problem, considering their prior experiences and learning from failure (e.g., Bryan et al., 2015 ), adjusting their reasoning and actions. In this context, it is essential to use alternative learning and teaching strategies that can awaken students to learn mathematics from a STEAM perspective through mathematical connections with real life and other disciplines, in particular focusing on ED through a problem-solving approach using authentic tasks (e.g., Diego-Mantecón et al., 2019 ). Since this is an innovative and recent approach in mathematics classes of all levels of schooling, mainly in elementary schools, we have to introduce these experiences in preservice teacher-training programs.
3 Methodology
This article describes a study that aims to understand and characterize the learning and reactions of future primary school teachers (6–12 years old) while solving and authentic problem in a STEAM context using ED. We also intended to identify the main difficulties and contents mobilized in the process. To conduct this research, we followed an exploratory qualitative and interpretative approach methodology (e.g., Creswell, 2009 ; Erickson, 1986 ; Miles & Huberman, 1994 ), because our aim is to understand the perspective and reactions of the participants to a particular situation, and not much is known about the situation that we want to examine.
The participants were 45 future teachers enrolled in an undergraduate course in primary education who attended a curricular unit of integrated mathematics, in the scope of didactics of mathematics. The majority of these future teachers (75%) were not interested in math, seeing it as a subject that they had to attend during their teacher training course to obtain their professional certificate, because the majority wants to work in the early ages of primary education, thinking they will only need to know the mathematics they will teach. In this sense, this curricular unit aims to give a different view of mathematics to captivate these students to learn mathematics themselves, so that they can teach it to their future pupils (e.g., Diego-Mantecón et al., 2019 ). We proposed innovative and active approaches (e.g., Math Trails with and without technology, Gallery Walk, Paper folding) as an alternative to the more traditional practices they contacted during their compulsory schooling. Hence, the work that was developed during the semester, before this didactical experience, focused on fundamental transversal skills, like the 4Cs, and taking into consideration the principles of active learning. It included teaching modules about problem-solving and problem-posing, creativity, reasoning, communication, and mathematical connections (e.g., within mathematics, with other disciplines, and with everyday life). This didactical experience happened during the module about problem-solving. This didactical experience happened during the module about problem-solving and encompassed the previously mentioned ideas by demanding the construction of a new product, a paper table, following the principles of ED. At first, participants did not see the connection with all they had done before, mainly to mathematics. We began with an introductory framework about the main ideas of STEAM education, presenting the ED cycle ( Figure 1 ) as a process that can improve the problem-solving skills of these future teachers/students. In this context, we proposed an authentic problem, the Paper Table Problem ( Figure 2 ).

The Paper Table Problem.
The experience was implemented during two classes in a total of 4 h, where the participants worked in groups of 3 or 4 elements. To solve the problem, we provided some materials: one cardboard sheet; eight publicity leaflets; one masking tape; one heavy book; one white sheet of paper (A2), and drawing supplies. They did not have access to more materials, even if they needed them. There were some instructions to follow, namely using the ED process cycle ( Figure 1 ) during the construction of the table. Students had access to some questions that could be asked at each of the different steps of the ED process cycle, adapted to the table problem, to aid them to get a prototype. It was intended that the students share their results as a group, so after the table construction, they had to create a poster, accompanied by the prototype, explaining all the work developed to reach the solution, characterizing each phase of the ED cycle, including their difficulties, and identifying the main concepts, ideas, and procedures of mathematics and physics, and work with their peers.
Data were collected in a holistic, descriptive, and interpretative manner during the classes of those preservice teachers, using several sources of data collection: participant observation; observational notes of the future teachers’ conversations, reactions, and interactions; documents, namely individual records and the group poster with the synthesis of the ED cycle and comments; artifacts (prototype of the table); and photos. To analyze the data, we used a qualitative and inductive approach, repeated to content analysis (Miles & Huberman, 1994 ), relying on written productions, artifacts, observations made, and field notes. We used an interactive process of analysis of data grounded not only in the collected data but also in the research problem and the theoretical framework, which we organized according to the following dimensions: (1) participants’ performance during the resolution of the table problem, the use of the ED cycle, and the construction of the poster; (2) STEAM contents identified by the participants , according to the subjects of the STEAM anagram; and (3) participants’ reactions during the didactical experience, focusing in particular on affective and behavioral features related to the table construction and the use of the ED cycle.
4 Results and Discussion
As we mentioned earlier, to achieve the solution to the problem, the participants had to follow the ED cycle, and they could only construct the prototype after making a sketch of the table, which they decided to do within the group.
Figure 3 shows images of the work developed by the students during the implementation of the first three steps of the ED cycle. All the students reacted to the challenge with some doubts at the beginning of the process because they had never solved a task of this nature and had some resistance to use the different steps of the ED cycle. After overcoming some of the initial difficulties, they began to identify the type of table they could construct.

Students’ work through the steps 1–2–3 of ED.
The discussions focused on which shape to use for the table legs, mainly identifying some properties of the figures and shapes that could be stronger. The drawing of the table model revealed itself to be of great difficulty for almost all the students. In Figure 4 , we can see that the first two images show the sketches of the two groups that drew 2D models, because they could not do it in 3D (images from the poster).

2D–3D sketches.
In the first image, it is difficult to identify what type of structure was proposed for the support of the tabletop. The second shows a top view of three 2D drawings, where we can clearly identify the position of the table legs. However, they reinforced their ideas by putting into words what each drawing meant (a table with eight cylindrical legs, a table with six cylindrical legs, and a table to be tested). The last two images show 3D drawings, where the first sketch did not clearly illustrate how the arrangement of the legs would be, but in the last one, we can understand the structure of the table clearly.
After deciding what kind of table the participants wanted to build, they began to look for the paper that would be used in the construction of the table’s legs. They made many attempts – some rolled the paper to make tubes (cylinders); other groups opted to construct solids, mainly prisms; they used tape to tie the paper and confirmed the length of the legs. Then, after getting these elements ready, the groups began to use them as isolated legs or joined some of them to obtain some structures to support the top of the table. After this work through the 4–5–6 steps, they tested the resistance of the table using the book’s weight (or even more books) ( Figure 5 ).

Students’ work through steps 4–5–6 of ED.
In some cases, on the first try, the table stood for some seconds but fell down when they put the book on it. These students had to revise the chosen model, the way they rolled the paper to get the tubes, or the way they connected the elements; others got a solid prototype table at the first attempt. This was the most difficult part of the construction for many groups. The use of tape allowed them to overcome some difficulties and strengthen the table legs. One group that used the legs in the shape of a triangle Indian tent, when testing the table with the book, concluded that the legs began to tilt and twist. The solution to overcome this fragility of the table legs was to triangulate the table legs because one of the students said: “I remember, from math, that the triangle is the more rigid shape” ( Figure 6 ).

Improvement of the stability of the table.
Most of the students made their structures stronger using their intuition, daily know-how, or trial-and-error strategy. They could not properly explain how certain concepts or principles strengthen the table, although everyone managed to build a table. All participants arrived at step 7, where Figure 7 shows some of the participants’ prototypes of the table.

Table prototypes through step 7 of ED.
Nevertheless, the participants were able to be successful and construct a table. Then, they began to create their poster, following the given previous instructions. In this step, they discussed: how to organize the information through the poster; then they had to revisit the ED cycle, thinking back on the work developed to describe their thoughts and processes in each of the ED steps. With the prototype in front of them, they began to look for the ideas, concepts, and procedures they used in the scope of mathematics and physics. They had some aesthetic concerns with the poster constructions, already shown during the construction of the table model, some more than others. To finalize the poster, they also had to describe their reactions to this experience.
We can see some of the posters in Figure 8 .

Some of the posters.
Our main preliminary results can be synthesized into the following ideas. These future teachers valued the experience because they had the opportunity to solve an authentic problem, which they had not yet done, in a collaborative way. They already had the opportunity to mobilize mathematical concepts mainly related to geometry (e.g., prisms, pyramids, cubes, angles, measurement, and symmetry) and physics contents (centers of mass and forces) in an integrated way. They exhibited great engagement, showing persistence and motivation in solving the problem, through many discussions, trials, and errors, but all were able to create a model according to the requested conditions. They mentioned that the model helped them think through the problem and execute their idea (model), although there were steps that they did not distinguish. They had attention to aesthetic features, not only in the preparation of the poster but also in the construction of the table prototype. Finally, they said that it was an experience they would like to repeat during their training and also use with their own pupils in the future.
However, some difficulties were identified by them and detected by us, namely: in drawing the sketch (3D); in the identification of some mathematical concepts; in the identification of some concepts of physics (one of the aspects observed was that, despite not verbalizing the physics and mathematical concepts, during the manipulation of the materials, it was concluded that these concepts were present in the way they built and in the way they positioned the book to test the table’s stability to support the book); in the mobilization of adequate scientific language in the reasoning underlying their decisions; in precisely justifying the need to improve the design/execution plan of the model; in the use/interpretation of the ED model. Most students did not follow the cycle in the presented order, starting with the manipulation of materials and then going back to contextualize the problem. Concluding, we did not expect that the preservice teachers had such narrow concepts of the sciences, much of them common sense, so these findings highlight the need for further scaffolding to prompt participants to use both sound scientific and mathematical knowledge. This is an important aspect to consider in the sense that they need to be aware of the content knowledge underlying the work with STEAM-related real problems or situations, building their confidence to work with their future students.
5 Conclusions
This experience gave an opportunity to these students, future teachers, to solve a real-life problem with simple materials, such as a sheet of paper. At the same time, it favored the integration of some disciplinary areas such as mathematics, science, and art, as well as the fundamentals of engineering, occasionally resorting to technology (Bryan et al., 2015 ; Cunningham & Hester, 2007 ; Diego-Mantecón et al., 2019 , 2022 ; Martín-Páez et al., 2019 ). Science, particularly physics, contributed to justify the balance and resistance of the table and the materials used. The technology was used spontaneously in the exploration phase during brainstorming. Engineering contributed through its design model to address the problem. The arts contributed to the aesthetics/design of the prototype and the elaboration of the poster mathematics contributed to solving the task, allowing us, e.g., to use problem-solving strategies and to understand the effect of using some geometric concepts (shapes and the idea of symmetry, measurements, and weight).
The implementation of the paper table problem evidenced that these future teachers were able to follow the ED cycle, imagining, designing, (re)building, and (re)testing. Some tried to skip the sketches due to their difficulties in representing 3D shapes or due to their immediate need to experiment with the materials. This experience, a challenge for these future teachers, increased their interest in mathematics and let them recognize that engineering has many ties to mathematics, in particular with problem-solving, that they study and use in class. However, there are some aspects to improve, like refining the materials used and proposing tasks where technology has more expression. Also, we need to have more studies that give us insights into the interdisciplinarity and discover a more predominant role of mathematics in these tasks/projects (e.g., Diego-Mantecón et al., 2019 , 2022 ). We were able to identify potentialities in the use of ED to solve this type of problems, like the positive engagement with the task and the possibility to establish connections between mathematics and physics, two subjects that students consider to be difficult. But we also recognize that it will be challenging for educators to look for tasks that are suitable for the elementary school level since we had some difficulties in finding adequate problems/projects for these school levels.
To conclude, these students, future teachers, said that they would like to have the opportunity to repeat this experience with other problems, so it will be a practice to implement this discipline in future courses. Thus, teacher education programs should include innovative experiences that stimulate preservice teachers’ knowledge, particularly solving the same tasks and using the same teaching and learning principles that they are expected to use with their own future students (e.g., Cooney & Krainer, 1996 ; Sullivan, Clarke, & Clarke, 2013 ; Vale & Barbosa, 2021 ; Vale, Pimentel, & Barbosa, 2018 ).
Funding information: This research received no specific grant from any funding agency, commercial or nonprofit sectors.
Conflict of interest: The authors state that there is no conflict of interest.
Barbosa, A., & Vale, I. (2018). As dobragens como uma estratégia de aprendizagem ativa [Paper folding as an active learning strategy]. In D. Alves, H. Pinto, I. Dias, M. Abreu, & R. Muñoz (Eds.), Atas da VII Conferência Internacional Investigação Práticas e Contextos em Educação (pp. 83–91). Leiria: Escola Superior de Educação e Ciências Sociais Instituto Politécnico de Leiria. Search in Google Scholar
Bryan, L. A., Moore, T. J., Johnson, C. C., & Roehrig, G. H. (2015). Integrated STEM education. In C. C. Johnson, E. E. Peters-Burton, & T. J. Moore (Eds.), STEM roadmap: A framework for integration (pp. 23–37). London: Taylor & Francis. 10.4324/9781315753157-3 Search in Google Scholar
Cooney, T., & Krainer, K. (1996). Inservice teacher mathematics education: The importance of listening. In A. Bishop, K. Clements, C. Keitel, J. Kilpatrick, & C. Laborde (Eds.), International handbook of mathematics education (pp. 1155–1185). Dordrecht: Kluwer Academic Press. 10.1007/978-94-009-1465-0_36 Search in Google Scholar
Creswell, J. W. (2009). Research design: Qualitative, quantitative and mixed methods approaches. Thousand Oaks: Sage Publications. Search in Google Scholar
Cunningham, C. M., & Hester, K. (2007). Engineering is elementary: An engineering and technology curriculum for children. Paper presented at ASSE Annual Conference and Exposition. HI. http://www.eie.org/sites/default/files/research_article/research_file/ac2007full8.pdf. Search in Google Scholar
Design Squad Global. (2019). Design Squad Global Inventing Green 12-week CLUB guide. WGBH. https://pbskids.org/designsquad/pdf/parentseducators/DSG_Inventing_Green_Club_Guide_6_Week.pdf. Search in Google Scholar
Diego-Mantecón, J. M., Ortiz-Laso, Z., & Blanco, T. F. (2022). Implementing STEM projects through the EDP to learn mathematics: The importance of teachers’ specialization. In Mathematics education in the age of artificial intelligence: How artificial intelligence can serve mathematical human learning (pp. 399–415). Cham: Springer International Publishing. 10.1007/978-3-030-86909-0_17 Search in Google Scholar
Diego-Mantecón, J. M., Arcera, O., Blanco, T. F., & Lavicza, Z. (2019). An engineering technology problem-solving approach for modifying student mathematics-related beliefs: Building a robot to solve a Rubik’s cube. International Journal for Technology in Mathematics Education, 26(2), 55–64. Search in Google Scholar
Edwards, S. (2015). Active learning in the middle grades. Middle School Journal, 46(5), 26–32. 10.1080/00940771.2015.11461922 Search in Google Scholar
Edwards, S., Kemp, A., & Page, C. (2014). The middle school philosophy: Do we practice what we preach or do we preach something different? Current Issues in Middle Level Education, 19(1), 13–19. Search in Google Scholar
English, L. D., & King, D. T. (2015). STEM learning through engineering design: Fourth-grade students’ investigations in aerospace. International Journal of STEM Education, 2(14), 1–18. 10.1186/s40594-015-0027-7 Search in Google Scholar
English, L. D., & King, D. (2019). STEM integration in sixth grade: Designing and constructing paper bridges. International Journal of Science and Mathematics Education, 17, 863–884. 10.1007/s10763-018-9912-0 . Search in Google Scholar
Erickson, F. (1986). Qualitative methods in research on teaching. In M. C. Wittrock (Ed.), Handbook of research on teaching (pp. 119–161). New York: Macmillan. Search in Google Scholar
Hannaford, C. (2005). Smart Moves: Why learning is not all in your head. Salt Lake City: Great River Books. Search in Google Scholar
Hannula, M. (2001). The metalevel of emotion-cognition interaction. In M. Ahtee, O. Björkqvist, E. Pehkonen, & V. Vatanen (Eds.), Research on mathematics and science education. From beliefs to cognition, from problem solving to understanding (pp. 55–65). Finland: University of Jyväskylä, Institute for Educational Research. Search in Google Scholar
Hiebert, J., Carpenter, T. P., Fennema, E., Fuson, K. C., Wearne, D., Murray, H., … Human, P. (1997). Making sense: Teaching and learning mathematics with understanding. Portsmouth, NH: Heinemann. Search in Google Scholar
Kramarski, B., & Mevarech, Z. (2014). Critical Maths for Innovative Societies- the role of metacognitive pedagogies. Paris: OECD. 10.1787/9789264223561-en Search in Google Scholar
Liljedahl, P. (2016). Building thinking classrooms: Conditions for problem solving. In P. Felmer, E. Pehkonen, & J. Kilpatrick (Eds.), Posing and solving mathematical problems (pp. 365–386). Switzerland: Springer. 10.1007/978-3-319-28023-3_21 Search in Google Scholar
Maass, K., Doorman, M., Jonker, V., & Wijers, M. (2019). Promoting active citizenship in mathematics teaching, ZDM, 51, 991–1003. 10.1007/s11858-019-01048-6 Search in Google Scholar
Martín-Páez, T., Aguilera, D., Perales-Palacios, F. J., & Vílchez-González, J. M. (2019). What are we talking about when we talk about STEM education? A review of literature. Science Education, 103, 799–822. 10.1002/sce.21522 Search in Google Scholar
Miles, M. B. & Huberman, A. M. (1994). Qualitative data analysis. Thousand Oaks: Sage Publications. Search in Google Scholar
NCTM. (2014). Principles to actions: Ensuring mathematical success for all. Reston/VA: NCTM. Search in Google Scholar
Prince, M. (2004). Does active learning work? A review of the research. Journal of Engineering Education, 93, 223–231. 10.1002/j.2168-9830.2004.tb00809.x Search in Google Scholar
Schleicher, A. (2016). Teaching excellence through professional learning and policy reform: Lessons from around the world. Paris: International Summit on the Teaching Profession, OECD Publishing. 10.1787/9789264252059-en Search in Google Scholar
Sriraman, B., & Freiman, V. (2009). Interdisciplinarity for the 21st century: Proceedings of the 3rd international symposium on mathematics and its connections to arts and sciences. The Montana Mathematics Enthusiast Monographs in Mathematics Education, Monograph, 11. Search in Google Scholar
Sullivan, P., Clarke, D., & Clarke, B. (2013). Teaching with tasks for effective mathematics learning. New York: Springer. 10.1007/978-1-4614-4681-1 Search in Google Scholar
Vale, I., & Barbosa, A. (2020). Mathematics & movement: The gallery walk strategy. In G. S. Carvalho, P. Palhares, F. Azevedo, & C. Parente (Eds.), Improving children’s learning and well-being (pp. 7–22). Braga: CIEC/Instituto de Educação. https://www.researchgate.net/publication/341763598_Mathematics_Movement_the_gallery_walk_strategy. Search in Google Scholar
Vale, I., & Barbosa, A. (2021). Posters: A tool to foster visual and communication skills through mathematical challenging tasks using a gallery walk. In Proceedings of EDULEARN21 Conference (pp. 8416–8425). 10.21125/edulearn.2021.1706 Search in Google Scholar
Vale, I., Pimentel, T., & Barbosa, A. (2018). The power of seeing in problem solving and creativity: An issue under discussion. In S. Carreira, N. Amado, & K. Jones (Eds.), Broadening the Scope of Research on Mathematical Problem Solving: A Focus on Technology, Creativity and Affect (pp. 243–272). Switzerland: Springer. 10.1007/978-3-319-99861-9_11 Search in Google Scholar
WEF. (2016). New Vision for Education: Fostering Social and Emotional Learning through Technology. http://www3.weforum.org/docs/WEF_New_Vision_for_Education.pdf. Search in Google Scholar
© 2023 the author(s), published by De Gruyter
This work is licensed under the Creative Commons Attribution 4.0 International License.
- X / Twitter
Supplementary Materials
Please login or register with De Gruyter to order this product.
Journal and Issue
Articles in the same issue.
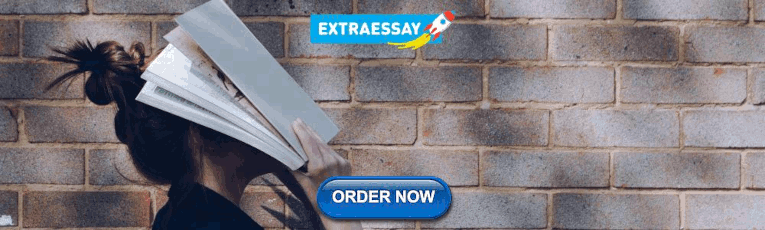
IMAGES
VIDEO
COMMENTS
Modelling, Synthesis and Problem Solving Strategies. Book • 1999. Authors: ... Modelling, Synthesis and Problem Solving Strategies. ... Introduction to Engineering Design is a completely novel text covering the basic elements of engineering design for structural integrity. Some of the most important concepts that students must grasp are those ...
Introduction to Engineering Design is a completely novel text covering the basic elements of engineering design for structural integrity. Some of the most important concepts that students must grasp are those relating to 'design thinking' and reasoning, and not just those that relate to simple theoretical and analytical approaches.
Introduction to Engineering Design is a completely novel text covering the basic elements of engineering design for structural integrity. Some of the most important concepts that students must grasp are those relating to 'design thinking' and reasoning, and not just those that relate to simple theoretical and analytical approaches. This is what will enable them to get to grips with *practical ...
It provides an overview of the engineering design process, emphasizing the integration of creativity and functionality in problem-solving. Key skills covered in this course include creative problem-solving techniques specific to design engineering, utilization of computer-aided design (CAD) and simulation tools, project management strategies ...
PART 1: MODELLING AND SYNTHESIS: Introducing Modelling and Synthesis for Structural Integrity Design against failure Design Synthesis of Generic Engineering Components Design of Mechanical Connections Review: Structural Integrity of Systems. PART 2: PROBLEM SOLVING STRATEGIES: AN ENGINEERING CULTURE: The Evolution of Design Problems Economic and Environmental Issues: The 'Big Picture'.
Understand Problem/Context Dene Design Criteria Solution Stage Brainstorm Solution Options Evaluate Solutions Prototype Solution Test Solution Figure 0.1: Engineering design process. By the end of this section, you should be equipped to: • Identify the goals of each step in the EDP, • Understand the role of the EDP in problem solving, and
Introduction to engineering design : modelling, synthesis and problem solving strategies / Andrew Samuel, John Weir. Authors Samuel, A. E. Publication 1999 ISBN 0585470669 Publication type Book. Ways to access this item. See if it's available through your library. Find your institution.
PART 2: PROBLEM SOLVING STRATEGIES: AN ENGINEERING CULTURE: The Evolution of Design Problems; Economic and Environmental Issues: The 'Big Picture' Summary: Introduction to Engineering Design is a completely novel text covering the basic elements of engineering design for structural integrity.
INTRODUCTION. Engineering design is the creative process of identifying needs and then devising a solution to fill those needs. This solution may be a product, a technique, a structure, a project, a method, or many other things depending on the problem. The general procedure for completing a good engineering design can be called the Engineering ...
This is what will enable them to get to grips with 'practical' design problems, and the starting point is thinking about problems in a 'decontructionist' sense. Full contents: Machine derived contents note: Table of contents for Introduction to engineering design : modelling, synthesis and problem solving strategies / Andrew Samuel, John Weir.
Modelling, Synthesis and Problem Solving Strategies . To Eva and Cathy . Introduction to Engineering Design: Modelling, Synthesis and Problem Solving Strategies Andrew Samuel John Weir ELSEVIER BU'FFERWORTH HEINEMANN AMSTERDAM 9 BOSTON 9 HEIDELBERG 9 LONDON 9 NEW YORK 9 OXFORD PARIS 9 SAN DIEGO ~ SAN FRANCISCO- SINGAPORE ~ SYDNEY 9 TOKYO ...
Introduction to Engineering Design is a completely novel text covering the basic elements of engineering design for structural integrity. Some of the most important concepts that students must grasp are those relating to 'design thinking' and reasoning, and not just those that relate to simple theoretical and analytical approaches. This is what will enable them to get to grips with *practical ...
The book has three parts. Part 1 - Understanding - is intended to provide an overview of some of the major findings as to what constitutes design synthesis, and some of its major infiuencing factors. Part 2 - Approaches - provides descriptions of some of the major prescriptive approaches to design synthesis that together infiu enced many of ...
Dym is the author of several books, includingEngineering Design: A Synthesis of Views(1994);Structural Modeling and Analysis(1997);Principles of Mathematical Modeling, Second Edition(2004); andEngineering Design: A Project-Based Introduction, Third Edition, coauthored with Patrick Little (2008). He has published more than 90 refereed journal ...
Introduction to engineering design : modelling, synthesis and problem solving strategies / Andrew Samuel, John Weir.
Introduction to Engineering Design: Modelling, Synthesis and Problem Solving Strategies | Andrew Samuel, John Weir | download on Z-Library | Download books for free. Find books. Support us in the fight for the freedom of knowledge Sign the petition Hide info. books search books;
Select search scope, currently: catalog all catalog, articles, website, & more in one search; catalog books, media & more in the Stanford Libraries' collections; articles+ journal articles & other e-resources
3.5 A Framework for Problem Solving. 3.6 How Much CO Does a Typical Passenger Car Produce? 3.7 Planning Larger Projects. 3.8 Heuristics. Problems. Part II MODEL-BASED DESIGN. 4 Laws of Nature and Theoretical Models. 4.1 Engineering Models. 4.2 Evolution of Theory. 4.3 Models of Motion. 4.4 Modeling the "Spring of Air". 4.5 The Birth of the ...
Introduction to Engineering Design: Modelling, Synthesis and Problem Solving Strategies | Andrew Samuel, John Weir | download on Z-Library | Download books for free. Find books Dukung kami dalam perjuangan kebebasan pengetahuan Menandatangani petisi Menyembunyikan
Engineering contributed through its design model to address the problem. The arts contributed to the aesthetics/design of the prototype and the elaboration of the poster mathematics contributed to solving the task, allowing us, e.g., to use problem-solving strategies and to understand the effect of using some geometric concepts (shapes and the ...
ÐÏ à¡± á> þÿ ~ þÿÿÿþÿÿÿ ...