Isolation and molecular characterization of five entomopathogenic nematode species and their bacterial symbionts from eastern Australia
- Published: 17 August 2021
- Volume 67 , pages 63–74, ( 2022 )
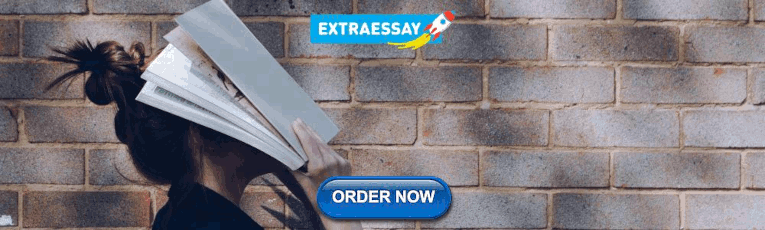
Cite this article
- Sitaram Aryal ORCID: orcid.org/0000-0002-4265-7663 1 ,
- Uffe N. Nielsen ORCID: orcid.org/0000-0003-2400-7453 1 ,
- Nanette H. Sumaya ORCID: orcid.org/0000-0002-2645-1120 2 ,
- Stefano De Faveri ORCID: orcid.org/0000-0001-5360-5570 3 ,
- Craig Wilson 4 &
- Markus Riegler ORCID: orcid.org/0000-0001-7363-431X 1
1024 Accesses
5 Citations
10 Altmetric
Explore all metrics
Entomopathogenic nematodes (EPNs) are used in biological control of pest insects but their potential may be limited by strain availability from different bioregions and effectiveness against specific pests. Here, we isolated and characterized EPNs and their symbiotic bacteria from Australia where their diversity is scarcely known. We collected 198 soil samples from citrus orchards, grasslands and forests across temperate, subtropical and tropical eastern Australia. EPNs were isolated by baiting with mealworm, greater wax moth and Queensland fruit fly, The Australia’s most significant horticultural pest. We obtained 36 isolates which, according to DNA sequence analyses, represented five species, Heterorhabditis bacteriophora , Heterorhabditis indica , Heterorhabditis marelatus , Heterorhabditis zealandica and Steinernema feltiae , including the first report of H. marelatus from Australia, and H. indica and H. zealandica from New South Wales. Thirty-five isolates were baited with mealworm, one with fruit fly, and none with wax moth. Heterorhabditis marelatus was recovered from forests, H. bacteriophora from citrus orchards, S. feltiae from citrus orchards and grasslands, H. indica and H. zealandica from all three habitats. According to bacterial DNA analyses, Photorhabdus heterorhabditis occurred in H. zealandica and a reference strain of H. bacteriophora , Photorhabdus laumondii in H. bacteriophora and H. marelatus , Photorhabdus tasmaniensis in H. indica and H. bacteriophora , and Photorhabdus namnaonensis in H. zealandica . Unexpectedly, Pseudomonas protegens and Delftia acidovorans were found in S. feltiae while its expected symbiont Xenorhabdus remained undetected, possibly due to our approach. The newly isolated EPNs should be tested as biological control agents against pest insects.
This is a preview of subscription content, log in via an institution to check access.
Access this article
Price includes VAT (Russian Federation)
Instant access to the full article PDF.
Rent this article via DeepDyve
Institutional subscriptions
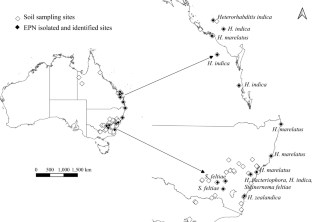
Similar content being viewed by others
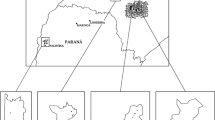
Entomopathogenic nematodes in agricultural areas in Brazil
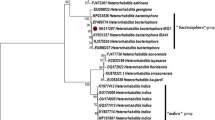
Molecular characterizations of the entomopathogenic nematodes, Heterorhabditis bacteriophora and Oscheius myriophilus from Iraq
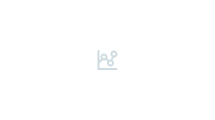
Global distribution of entomopathogenic nematodes, Steinernema and Heterorhabditis
Data availability.
All sequence data have been deposited in GenBank (NCBI). All other data are contained within the manuscript and supplementary material.
Abate BA, Wingfield MJ, Slippers B, Hurley BP (2017) Commercialisation of entomopathogenic nematodes: should import regulations be revised? Biocontrol Sci Tech 27:149–168
Google Scholar
Akhurst R, Bedding R (1986) Natural occurrence of insect pathogenic nematodes (Steinernematidae and Heterorhabditidae) in soil in Australia. Aust J Entomol 25:241–244
Bedding R, Akhurst R (1975) A simple technique for the detection of insect paristic rhabditid nematodes in soil. Nematologica 21:109–110
Bedding RA, Tyler S, Rochester N (1996) Legislation on the introduction of exotic entomopathogenic nematodes into Australia and New Zealand. Biocontrol Sci Tech 6:465–476
Boemare NE, Akhurst RJ, Mourant RG (1993) DNA relatedness between Xenorhabdus spp. (Enterobacteriaceae), symbiotic bacteria of entomopathogenic nematodes, and a proposal to transfer Xenorhabdus luminescen s to a new genus, Photorhabdu s gen. nov. Int J Syst Evol Microbiol 43:249–255
CAS Google Scholar
Boff M, Wiegers G, Smits P (2000) Influences of host size and host species on the infectivity and development of Heterorhabditis megidis (strain NLH-E87. 3). BioControl 45:469–482
Bonifassi E, Fischer-Le Saux M, Boemare N, Lanois A, Laumond C, Smart G (1999) Gnotobiological study of infective juveniles and symbionts of Steinernema scapterisci : a model to clarify the concept of the natural occurrence of monoxenic associations in entomopathogenic nematodes. J Invertebr Pathol 74:164–172
CAS PubMed Google Scholar
Brillard J, Duchaud E, Boemare N, Kunst F, Givaudan A (2002) The PhlA hemolysin from the entomopathogenic bacterium Photorhabdus luminescens belongs to the two-partner secretion family of hemolysins. J Bacteriol 184:3871–3878
CAS PubMed PubMed Central Google Scholar
Campos-Herrera R, Gomez-Ros JM, Escuer M, Cuadra L, Barrios L, Gutiérrez C (2008) Diversity, occurrence, and life characteristics of natural entomopathogenic nematode populations from La Rioja (Northern Spain) under different agricultural management and their relationships with soil factors. Soil Biol Biochem 40:1474–1484
Canhilal R, Waeyenberge L, Yüksel E, Koca AS, Deniz Y, İmren M (2017) Assessment of the natural presence of entomopathogenic nematodes in Kayseri soils, Turkey. Egypt J Biol Pest Control 27:237–244
Ciche TA, Darby C, Ehlers R-U, Forst S, Goodrich-Blair H (2006) Dangerous liaisons: the symbiosis of entomopathogenic nematodes and bacteria. Biol Control 38:22–46
Cimen H, Půža V, Nermuť J, Hatting J, Ramakuwela T, Faktorova L, Hazir S (2016) Steinernema beitlechemi n. sp., a new entomopathogenic nematode (Nematoda: Steinernematidae) from South Africa. Nematology 18:439–453
De Brida AL, Rosa JMO, De Oliveira CMG, e Castro BMDC, Serrão JE, Zanuncio JC, Leite LG, Wilcken SRS (2017) Entomopathogenic nematodes in agricultural areas in Brazil. Sci Rep 7:45254
PubMed PubMed Central Google Scholar
Dominiak BC, Mapson R (2017) Revised distribution of Bactrocera tryoni in eastern Australia and effect on possible incursions of Mediterranean fruit fly: development of Australia’s eastern trading block. J Econ Entomol 110:2459–2465
PubMed Google Scholar
Dowds BCA, Peters A (2002) Virulence mechanisms. In: Gaugler R (ed) Entomopathogenic nematology. CABI Publishing, Wallingford, pp 79–98
Dreyer J, Malan AP, Dicks LM (2017) Three novel Xenorhabdus - Steinernema associations and evidence of strains of X. khoisanae switching between different clades. Curr Microbiol 74:938–942
Fischer-Le Saux M, Mauléon H, Constant P, Brunel B, Boemare N (1998) PCR-ribotyping of Xenorhabdus and Photorhabdus isolates from the Caribbean region in relation to the taxonomy and geographic distribution of their nematode hosts. Appl Environ Microbiol 64:4246–4254
Fletcher BS (1974) The ecology of a natural population of the Queensland fruit fly, Dacus tryoni . VI. Seasonal changes in fruit fly numbers in the areas surrounding the orchard. Aust J Zool 22:353–363
Godjo A, Afouda L, Baimey H, Decraemer W, Willems A (2018) Molecular diversity of Photorhabdus and Xenorhabdus bacteria, symbionts of Heterorhabditis and Steinernema nematodes retrieved from soil in Benin. Arch Microbiol 200:589–601
Grewal PS, Ehlers R-U, Shapiro-Ilan DI (2005) Nematodes as biocontrol agents. CABI Publishing, Wallingford
Griffin CT, Boemare NE, Lewis EE (2005) Biology and behaviour. In: Grewal PS, Ehlers R-U, Shapiro-Ilan DI (eds) Nematodes as biocontrol agents. CABI Publishing, Wallingford, pp 47–64
Hominick W (2002) Biogeography. In: Gaugler R (ed) Entomopathogenic nematology. CABI Publishing, Wallingford, pp 115–144
Hoy CW, Grewal PS, Lawrence JL, Jagdale G, Acosta N (2008) Canonical correspondence analysis demonstrates unique soil conditions for entomopathogenic nematode species compared with other free-living nematode species. Biol Control 46:371–379
Hulthen AD, Clarke AR (2006) The influence of soil type and moisture on pupal survival of Bactrocera tryoni (Froggatt) (Diptera: Tephritidae). Aust J Entomol 45:16–19
Ishibashi N, Kondo E (1986) Steinernema feltiae (DD-136) and S. glaseri : persistence in soil and bark compost and their influence on native nematodes. J Nematol 18:310–316
James M, Malan AP, Addison P (2018) Surveying and screening South African entomopathogenic nematodes for the control of the Mediterranean fruit fly, Ceratitis capitata (Wiedemann). Crop Prot 105:41–48
Kanga FN, Waeyenberge L, Hauser S, Moens M (2012) Distribution of entomopathogenic nematodes in Southern Cameroon. J Invertebr Pathol 109:41–51
Kaya HK, Stock SP (1997) Techniques in insect nematology. In: Lacey LA (ed) Manual of techniques in insect pathology. Academic Press, Cambridge, pp 281–324
Koppenhöfer AM, Fuzy EM (2003) Steinernema scarabaei for the control of white grubs. Biol Control 28:47–59
Koppenhöfer AM, Fuzy EM (2006) Effect of soil type on infectivity and persistence of the entomopathogenic nematodes Steinernema scarabaei , Steinernema glaseri , Heterorhabditis zealandica , and Heterorhabditis bacteriophora . J Invertebr Pathol 92:11–22
Kour S, Khurma U, Brodie G, Hazir S (2020) Natural occurrence and distribution of entomopathogenic nematodes (Steinernematidae, Heterorhabditidae) in Viti Levu, Fiji Islands. J Nematol 52:1–17
Kumar S, Stecher G, Li M, Knyaz C, Tamura K (2018) MEGA X: molecular evolutionary genetics analysis across computing platforms. Mol Biol Evol 35:1547–1549
Kung S-P, Gaugler R, Kaya HK (1990) Influence of soil pH and oxygen on persistence of Steinernema spp. J Nematol 22:440–445
Langford EA, Nielsen UN, Johnson SN, Riegler M (2014) Susceptibility of Queensland fruit fly, Bactrocera tryoni (Froggatt) (Diptera: Tephritidae), to entomopathogenic nematodes. Biol Control 69:34–39
Liu J, Berry RE (1996) Heterorhabditis marelatus n. sp. (Rhabditida: Heterorhabditidae) from Oregon. J Invertebr Pathol 67:48–54
Liu J, Berry RE, Poinar GO (1998) Taxonomic comments on the genus Steinernema (Nematoda: Steinernematidae): specific epithets and distribution record. Nematologica 44:321–322
Machado RAR, Wüthrich D, Kuhnert P, Arce CCM, Thönen L, Ruiz C, Zhang X, Robert CAM, Karimi J, Kamali S (2018) Whole-genome-based revisit of Photorhabdus phylogeny: proposal for the elevation of most Photorhabdus subspecies to the species level and description of one novel species Photorhabdus bodei sp. nov., and one novel subspecies Photorhabdus laumondii subsp. clarkei subsp. nov. Int J Syst Evol Microbiol 68:2664–2681
Machado RA, Muller A, Ghazal SM, Thanwisai A, Pagès S, Bode HB, Hussein MA, Khalil KM, Tisa LS (2021) Photorhabdus heterorhabditis subsp. aluminescens subsp. nov., Photorhabdus heterorhabditis subsp. heterorhabditis subsp. nov., Photorhabdus australis subsp. thailandensis subsp. nov., Photorhabdus australis subsp. australis subsp. nov., and Photorhabdus aegyptia sp. nov. isolated from Heterorhabditis entomopathogenic nematodes. Int J Syst Evol Microbiol 71:004610
Malan AP, Nguyen KB, Addison MF (2006) Entomopathogenic nematodes (Steinernematidae and Heterorhabditidae) from the southwestern parts of South Africa. Afr Plant Prot 12:65–69
Malan AP, Knoetze R, Tiedt L (2014) Heterorhabditis noenieputensis n. sp. (Rhabditida: Heterorhabditidae), a new entomopathogenic nematode from South Africa. J Helminthol 88:139–151
Maneesakorn P, An R, Daneshvar H, Taylor K, Bai X, Adams BJ, Grewal PS, Chandrapatya A (2011) Phylogenetic and cophylogenetic relationships of entomopathogenic nematodes (Heterorhabditis: Rhabditida) and their symbiotic bacteria ( Photorhabdus : Enterobacteriaceae). Mol Phylogenetics Evol 59:271–280
McMullen JG, Peterson BF, Forst S, Blair HG, Stock SP (2017) Fitness costs of symbiont switching using entomopathogenic nematodes as a model. BMC Evol Biol 17:100
Ogier J-C, Pagès S, Frayssinet M, Gaudriault S (2020) Entomopathogenic nematode-associated microbiota: from monoxenic paradigm to pathobiome. Microbiome 8:25
Orozco RA, Lee M-M, Stock SP (2014) Soil sampling and isolation of entomopathogenic nematodes (Steinernematidae, Heterorhabditidae). J Vis Exp 89:e52083
Poinar GO (1975) Description and biology of a new insect parasitic rhabditoid, Heterorhabditis bacteriophora n. gen., n. sp. (Rhabditida; Heterorhabditidae n. fam.). Nematologica 21:463–470
Poinar GO (1990) Taxonomy and biology of Steinernematidae and Heterorhabditidae. In: Gaugler R, Kaya HK (eds) Entomopathogenic nematodes in biological control. CRC Press, Boca Raton, Florida, pp 23–61
Saitou N, Nei M (1987) The neighbor-joining method: a new method for reconstructing phylogenetic trees. Mol Biol Evol 4:406–425
Solomon A, Glazer I (1999) Desiccation survival of the entomopathogenic nematode Steinernema feltiae : induction of anhydrobiosis. Nematology 1:61–68
Stock SP, Pryor BM, Kaya HK (1999) Distribution of entomopathogenic nematodes (Steinernematidae and Heterorhabditidae) in natural habitats in California, USA. Biodivers Conserv 8:535–549
Strauch O, Oestergaard J, Hollmer S, Ehlers R-U (2004) Genetic improvement of the desiccation tolerance of the entomopathogenic nematode Heterorhabditis bacteriophora through selective breeding. Biol Control 31:218–226
Stuart RJ, Barbercheck ME, Grewal PS (2015) Entomopathogenic nematodes in the soil environment: distributions, interactions and the influence of biotic and abiotic factors. In: Campos-Herrera R (ed) Nematode pathogenesis of insects and other pests. Sustainability in plant and crop protection. Springer, Cham, pp 97–137
Susurluk A, Ehlers R-U (2008) Field persistence of the entomopathogenic nematode Heterorhabditis bacteriophora in different crops. BioControl 53:627–641
Tailliez P, Pages S, Ginibre N, Boemare N (2006) New insight into diversity in the genus Xenorhabdus , including the description of ten novel species. Int J Syst Evol Microbiol 56:2805–2818
Upadhyay A, Mohan S (2021) Bacillus subtilis and B. licheniformis isolated from Heterorhabditis indica infected Apple Root Borer ( Dorysthenes huegelii ) suppresses nematode production in Galleria mellonella . Acta Parasitol. https://doi.org/10.1007/s11686-021-00366-8
Article PubMed Google Scholar
Download references
Acknowledgements
We thank Geraldine Tilden for technical support with fruit fly rearing, Alexander Robertson, Giles Ross, Alihan Katlav and Kylie Baker for help with field sampling, Michael Duncan for supply of wax moths and bees wax, and Roy Akhurst and Ian Broughton for advice.
This research was supported by the Australian Research Council Industrial Transformation Training Centre (ARC-ITTC) Fruit Fly Biosecurity Innovation (IC150100026), with a PhD scholarship to SA, and the Department of Agriculture, Water and the Environment’s Strengthening Australia’s Fruit Fly System Research Program; project: A national biocontrol program to manage pest fruit flies in Australia (4-EKSH327).
Author information
Authors and affiliations.
Hawkesbury Institute of the Environment, Western Sydney University, Penrith, NSW, 2751, Australia
Sitaram Aryal, Uffe N. Nielsen & Markus Riegler
Department of Biological Sciences, College of Science and Mathematics, Mindanao State University-Iligan Institute of Technology, Iligan City, Philippines
Nanette H. Sumaya
Department of Agriculture and Fisheries (DAF), Mareeba, QLD, Australia
Stefano De Faveri
Ecogrow Environment Pty Ltd., P.O. Box 241, Westgate, NSW, 2048, Australia
Craig Wilson
You can also search for this author in PubMed Google Scholar
Contributions
SA, MR and UNN conceptualized and designed the experimental work. SA and MR collected material with support of SDF. CW provided additional material. SA performed the experiments, collected and analyzed the data, under guidance of MR and UNN, and advice of NHS and CW. MR was responsible for research funding. SA wrote the manuscript together with MR and UNN and with input of all other authors. All authors agree with the submission of the manuscript.
Corresponding authors
Correspondence to Sitaram Aryal or Markus Riegler .
Ethics declarations
Conflict of interest.
The authors do not have a conflict of interest, except that CW works at Ecogrow, a company that supplied three EPN isolates which were characterised as part of this study.
Additional information
Handling Editor: Ralf-Udo Ehlers
Supplementary Information
Below is the link to the electronic supplementary material.
Supplementary file1 (DOCX 614 kb)
Rights and permissions.
Reprints and permissions
About this article
Aryal, S., Nielsen, U.N., Sumaya, N.H. et al. Isolation and molecular characterization of five entomopathogenic nematode species and their bacterial symbionts from eastern Australia. BioControl 67 , 63–74 (2022). https://doi.org/10.1007/s10526-021-10105-7
Download citation
Received : 23 March 2021
Accepted : 05 August 2021
Published : 17 August 2021
Issue Date : February 2022
DOI : https://doi.org/10.1007/s10526-021-10105-7
Share this article
Anyone you share the following link with will be able to read this content:
Sorry, a shareable link is not currently available for this article.
Provided by the Springer Nature SharedIt content-sharing initiative
- Bactrocera tryoni
- Tenebrio molitor
- 16S rRNA gene
- D2-D3 region
- Phylogenetic analysis
- Find a journal
- Publish with us
- Track your research
- Research article
- Open access
- Published: 13 June 2019
Improved phylogenomic sampling of free-living nematodes enhances resolution of higher-level nematode phylogeny
- Ashleigh B. Smythe 1 ,
- Oleksandr Holovachov 2 &
- Kevin M. Kocot ORCID: orcid.org/0000-0002-8673-2688 3
BMC Evolutionary Biology volume 19 , Article number: 121 ( 2019 ) Cite this article
11k Accesses
51 Citations
23 Altmetric
Metrics details
Nematodes are among the most diverse and abundant metazoans on Earth, but research on them has been biased toward parasitic taxa and model organisms. Free-living nematodes, particularly from the clades Enoplia and Dorylaimia, have been underrepresented in genome-scale phylogenetic analyses to date, leading to poor resolution of deep relationships within the phylum.
We supplemented publicly available data by sequencing transcriptomes of nine free-living nematodes and two important outgroups and conducted a phylum-wide phylogenomic analysis including a total of 108 nematodes. Analysis of a dataset generated using a conservative orthology inference strategy resulted in a matrix with a high proportion of missing data and moderate to weak support for branching within and placement of Enoplia. A less conservative orthology inference approach recovered more genes and resulted in higher support for the deepest splits within Nematoda, recovering Enoplia as the sister taxon to the rest of Nematoda. Relationships within major clades were similar to those found in previously published studies based on 18S rDNA.
Conclusions
Expanded transcriptome sequencing of free-living nematodes has contributed to better resolution among deep nematode lineages, though the dataset is still strongly biased toward parasites. Inclusion of more free-living nematodes in future phylogenomic analyses will allow a clearer understanding of many interesting aspects of nematode evolution, such as morphological and molecular adaptations to parasitism and whether nematodes originated in a marine or terrestrial environment.
Nematodes are ubiquitous and diverse metazoans that are found free-living in nearly every terrestrial and aquatic habitat and parasitizing most animals and plants. Fewer than 30,000 species have been described, but the actual diversity of the phylum may be closer to 1 million species [ 1 ]. Despite estimates that at least half of all nematodes are free-living [ 1 , 2 ], most research has focused on parasitic nematodes of medical and agricultural importance. Particularly neglected are the free-living marine nematodes, with only around 6900 species described [ 3 ] and no genomes published to date [ 4 ]. Of significance, free-living nematodes are generally the most abundant and diverse metazoans of marine sediments [ 5 , 6 , 7 , 8 ] where they are important as decomposers, predators, food for higher trophic levels [ 9 ], and as bioindicators for climate change and ecological disturbance [ 10 , 11 , 12 ].
Despite the importance of nematodes as free-living animals and as parasites of humans, livestock, and crops, and despite more than a century of intensive research, certain aspects of their origin and early evolution, such as the branching order near the root of Nematoda, are not yet fully understood [ 13 , 14 ]. Nematode evolutionary history is particularly interesting because of the diversity of niches they occupy – ranging from the blood and tissues of vertebrate and invertebrate animals, unicellular eukaryotes, all parts of plants, virtually every terrestrial habitat, and all aquatic environments including deep-sea hydrothermal vent communities – is unrivaled in Metazoa [ 15 , 16 , 17 , 18 ]. Thus, resolving nematode phylogeny, especially the branching order close to the root of the nematode tree, will not only improve our understanding of the origin of economically important groups, but will provide a phylogenetic framework for understanding the underlying key characters (e.g., genomic modifications) corresponding to different nematode lifestyles, advancing all aspects of nematology, from basic evolutionary biology to pathogen control and drug development [ 19 ].
Morphology-based hypotheses of higher-level nematode relationships (reviewed by [ 17 , 20 , 21 , 22 ]) placed emphasis on the presence or absence of a lateral canal excretory system and a number of esophageal features [ 23 , 24 ]. These characters were interpreted as evidence of two major lineages: a primarily terrestrial (but also including many plant and animal parasites) grouping called Secernentea, and a primarily aquatic grouping called Adenophorea. Subsequent morphological investigations by Andrássy [ 25 ] and Malakhov [ 26 ] distinguished three main lineages, elevating the primarily aquatic Enoplia and Chromadoria out of Adenophorea and re-classifying most Secernentea as Rhabditia.
The first molecular phylogenetic hypothesis for Nematoda used 18S rDNA [ 27 ] and differed substantially from previous morphology-based hypotheses of nematode phylogeny (e.g. [ 24 ]). This and subsequent analyses based on 18S have led to the recognition of three major lineages of nematodes: Dorylaimia (Clade I), Enoplia (Clade II), and Chromadoria, which consists of Spirurina (Clade III), Tylenchina (Clade IV), Rhabditina (Clade V), Plectida, Araeolaimida, Monhysterida, Desmodorida, and Chromadorida [ 17 , 21 , 22 , 27 , 28 , 29 ]. Dorylaimia includes many free-living soil nematodes and plant parasites, but also vertebrate parasites such as Trichinella, whipworms, and Dioctophyme . Enoplia primarily consists of free-living aquatic nematodes, but also several lineages of soil nematodes and virus-transmitting plant pests (such as stubby root nematodes). Chromadoria includes a wide diversity of free-living aquatic nematodes but also familiar animal parasites (e.g. Ascaris , hookworms, and Dirofilaria ), plant parasites (e.g. cyst and root knot nematodes), and the model organism Caenorhabditis elegans .
Enoplia has generally been thought to represent the sister group to all remaining nematodes [ 7 , 30 ] because of the presence of presumably ancestral developmental features, which are common in other animal phyla but not seen in other lineages of nematodes thus far investigated. These include indeterminate development [ 31 , 32 , 33 ] and retention of the nuclear envelope in mature spermatozoa (other nematodes investigated to date have determinate development and spermatozoa that lose the nuclear envelope upon maturation [ 34 ]). As other metazoan lineages are thought to have marine origins, nematodes have traditionally been assumed to have evolved in the marine environment [ 24 , 26 , 35 ]. Thus the primarily marine habits of Enoplia, combined with their presumed ancestral developmental features, have led to them being viewed as the earliest-branching nematode lineage [ 7 , 30 ]. On the other hand, De Ley and Blaxter [ 22 ] suggested the possibility of a terrestrial origin of nematodes with the sister group to all other nematodes being the taxon least represented in the marine environment, Dorylaimia. Ribosomal DNA-based studies have been unable to resolve the branching order among these deepest branches within Nematoda. Even studies focused on improved representation of diverse marine free-living nematodes [ 7 , 36 ] failed to find resolution at the base of the nematode tree, suggesting that additional molecular markers are needed to resolve deep nematode phylogeny.
Recently, phylogenomic studies employing dozens to hundreds of nuclear protein-coding genes have addressed questions of nematode evolution, but taxon sampling in these studies has largely built on publicly available genome and transcriptome datasets [ 4 , 37 , 38 , 39 , 40 , 41 ]. Until now, phylogenomic analyses of Nematoda have focused on parasitic taxa and model Caenorhabditis spp., with little or no representation of other free-living nematodes. For example, Blaxter and Koutsovoulos [ 40 ] and Koutsovoulos [ 41 ] curated the largest phylogenomic datasets for Nematoda to date but included only a single member of Enoplia in their studies. The latest comparative phylogenomic study focusing on parasitic worms included a handful of free-living nematodes (mostly model organisms), but no representatives of Enoplia or early branching Chromadoria [ 42 ]. Likewise, phylogenetic analyses based on mitochondrial genomes have never included representatives of Enoplia [ 43 , 44 , 45 , 46 ] because the mitochondrial genome has not yet been sequenced for any member of this clade.
Here, we have assembled the largest and most diverse phylogenomic dataset for Nematoda to date with expanded transcriptome representation for previously undersampled free-living nematode taxa. Leveraging this dataset, we re-examine relationships among early-branching clades and provide a robustly resolved and expanded phylogenetic framework for Nematoda.
Publicly available nematode and outgroup genomes and transcriptomes were supplemented with new transcriptomes from nine free-living nematodes, one nematomorph, and one kinorhynch for a total of 131 taxa sampled (Table 1 , Additional file 2 : Tables S1-S2). Building on an established phylogenomic data processing pipeline [ 47 ], we assembled two datasets using two different sequence selection strategies (see Methods ). The first strategy used a strict orthology inference approach that refines initial orthology inference made by HaMStR [ 48 ] with PhyloTreePruner [ 49 ]. This strategy resulted in a dataset with 931 genes totalling 298,009 amino acids in length with 84.67% missing data. The second strategy employed SCaFoS [ 50 ] to select the best sequence for each taxon in the HaMStR output. The SCaFoS strategy resulted in a dataset with 1025 genes totalling 321,951 amino acids in length with 35.01% missing data.
Our results based on the matrix assembled with the more conservative PhyloTreePruner orthology inference strategy but with a higher proportion of missing data (Fig. 1 ) strongly support nematode monophyly (IQ-TREE / RAxML bootstrap support, bs = 100%/100%), and subsequent branching, with Enoplia being monophyletic (as previously recovered [ 7 , 51 ]), and the sister clade to Dorylaimia and Chromadoria. Enoplida+Triplonchida was moderately supported (bs = 88%/74%). However, Enoplida was paraphyletic with respect to Triplonchida, a single representative of which, Tobrilus sp., was included as an ingroup. Dorylaimia and Chromadoria were recovered as sister taxa with strong support in the IQ-TREE analysis (bs = 99%) and moderate support in the RAxML analysis (bs = 80%).
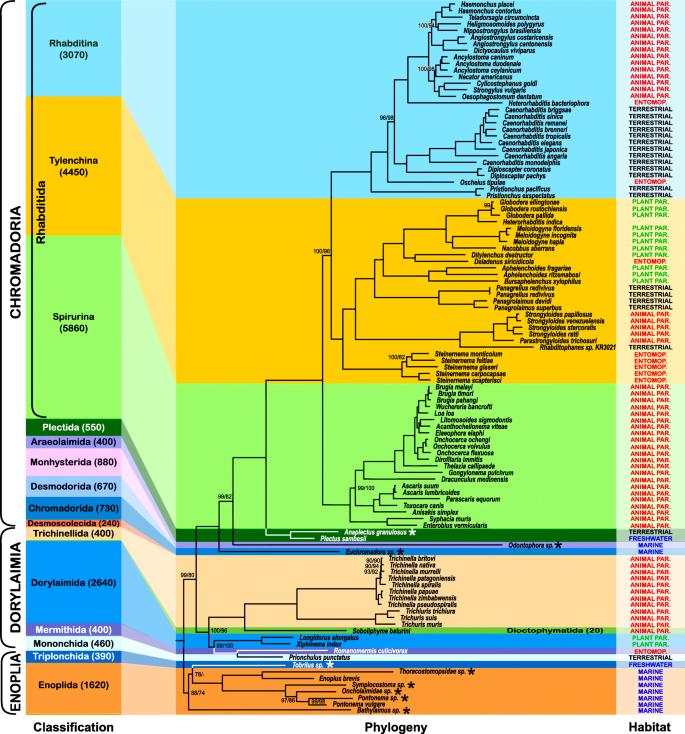
Phylogeny of Nematoda based on the IQ-TREE maximum likelihood analysis of the PhyloTreePruner dataset. “Classification” bar on the left side serves as a scale and represents the relative known taxonomic diversity of different taxa within Nematoda: the height of each colored bar is proportional to a number of known species (also given in the brackets after each taxon name), with the height of the entire multicolored background rectangle equal to 100% of known nematode diversity. IQ-TREE / RAxML bootstrap support values < 100% are shown. “Habitat” describes the lifestyle for each analysed species, such as animal parasitic (animal par.), plant parasitic (plant par.), entomopathogenic or entomoparasitic (entomop.), free-living freshwater (freshwater), terrestrial (terrestrial) and marine (marine). Newly generated transcriptomes are marked with an asterisk
Dorylaimia was strongly supported (bs = 100%/96%). This clade was primarily represented by members of the animal parasitic Trichinellida ( Trichinella and Trichuris ), which was also strongly supported as monophyletic (bs =100%/100%). Dorylaimida, which was represented by the virus-transmitting plant pests Longidorus elongatus and Xiphinema index , was also strongly supported as monophyletic (bs =100%/100%). Monophyly could not be tested for the remaining three orders represented by just one taxon each: Mononchida (represented by Prionchulus punctatus ), Mermithida (represented by Romanomermis culicivorax ), and Dioctophymatida (represented by Soboliphyme baturini ). Mermithida was recovered as the sister to Mononchida with maximal support.
Chromadoria was strongly supported (bs =100%/100%) with the sole representative of Chromadorida ( Euchromadora sp.) sister to a well-supported (bs = 99%/82%) clade of all other Chromadoria with Odontophora sp., the single representative of Araeolaimida, at the base. The two sampled representatives of Plectida ( Plectus sambesii and Anaplectus granulosus ) were recovered in a clade (bs =100%/100%) sister to Rhabditida. Rhabditida includes most described species of Chromadoria, and also most of the currently available transcriptomes and genomes. It received maximal support as did its three subclades: Spirurina, Tylenchina, and Rhabditina. Relationships within the major clades of Rhabditida were also consistently strongly supported. All genera for which monophyly was testable (i.e., those with more than one representative available for study), were recovered monophyletic, with the exception of Heterorhabditis . Heterorhabditis bacteriophora was strongly supported as sister to a clade composed of Trichostrongylidae and Ancylostomatidae within Rhabditina (as expected), while a species identified as H. indica was strongly supported as the sister taxon of Globodera spp. in Tylenchina.
Examination of the Heterorhabditis indica dataset [ 52 ] revealed that this organism was incorrectly identified or mislabelled – partial sequences of the nuclear ribosomal operon mined from the H. indica transcriptome assembly show high similarity to reference sequences from various species of the genera Heterodera and Globodera (Hoplolaimidae, Tylenchina), and not Heterorhabditis (Heterorhabditidae, Rhabditina). This is further confirmed by the results of our tree-based taxonomy assignment using the 18S rDNA gene fragment (Additional file 1 : Figure S1). Unfortunately, these partial sequences mined from the transcriptome of H. indica are relatively short, one with only 588 bases of the 5′ end of 18S rDNA and the other with just 863 bases of the 5′ end of 28S rDNA. They do not contain enough phylogenetically informative sites to ensure species-level identification.
Because of the high amount of missing data (84.67%) in the dataset assembled using PhyloTreePruner, we also used a less conservative orthology inference approach that did not employ an additional tree-based orthology confirmation after initial HaMStR orthology inference. This resulted in a larger and much more complete dataset with 1026 genes totalling 321,951 amino acids in length with 64.99% matrix completeness. Analysis of this SCaFoS-based dataset resulted in a nearly identical branching order as that of the PhyloTreePruner-based dataset (Fig. 2 ). Whereas support for Enoplia was weak in the analysis of the PhyloTreePruner-based dataset, analysis of this dataset recovered Enoplia monophyletic and sister to the rest of Nematoda with maximal support. Tobrilus sp. (Triplonchida) was recovered sister to Enoplida with maximal support and Bathylaimus sp. was recovered sister to all other Enoplida with maximal support, which is in agreement with 18S rDNA-based analyses by van Megen et al. [ 53 ], Bik et al. [ 7 ], and Smythe [ 51 ]. Relationships within Dorylaimia were strongly supported and identical to the results based on the PhyloTreePruner dataset with the exception of relationships among Trichinella nativa , T. britovi , and T. murrelli. Likewise, relationships within Chromadoria were nearly identical; the one difference was placement of Oscheius tipulae , which was recovered sister to Rhabditomorpha sensu De Ley & Blaxter, 2004 [ 22 ] in the analysis of the PhyloTreePruner dataset and sister to Strongyloidea sensu De Ley & Blaxter, 2004 [ 22 ] in the analysis of the SCaFoS dataset.
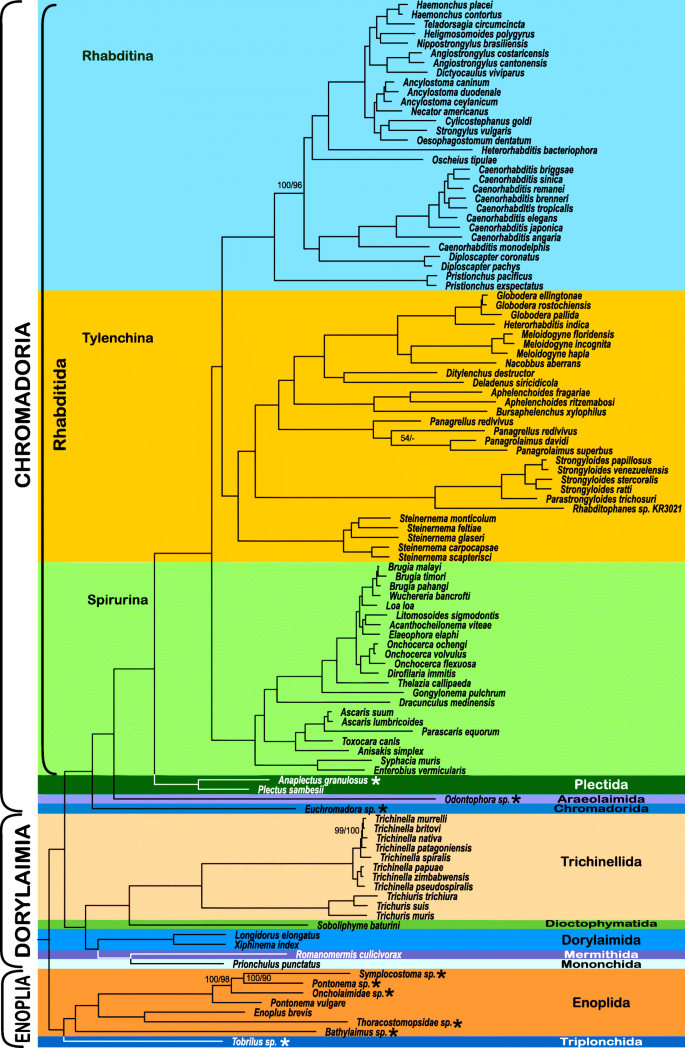
Phylogeny of Nematoda based on the IQ-TREE maximum likelihood analysis of the SCaFoS dataset. IQ-TREE / RAxML bootstrap support values < 100% are shown. Newly generated transcriptomes are marked with an asterisk
With respect to higher-level ecdysozoan (Fig. 3 ) relationships, both analyses recovered Scalidophora (represented by Priapulida + Kinorhyncha) monophyletic and sister to the rest of Ecdysozoa with strong support. IQ-TREE analysis of the PhyloTreePruner dataset recovered Onychophora sister to Arthropoda with strong support (bs = 98%) while the RAxML analysis had only moderate support for this placement (bs = 78%). However, analyses of the SCaFoS dataset recovered Onychophora sister to all non-scalidophoran ecdysozoans with similar levels of support (bs = 100%/82%). IQ-TREE analyses recovered Tardigrada sister to Nematoda with moderate to strong support (bs = 84–97%) whereas RAxML analyses recovered Tardigrada + Nematomorpha sister to Nematoda. This was strongly supported in the analysis of the SCaFoS dataset (bs = 100%) but weakly supported in the analysis of the PhyloTreePruner dataset (bs = 66%).
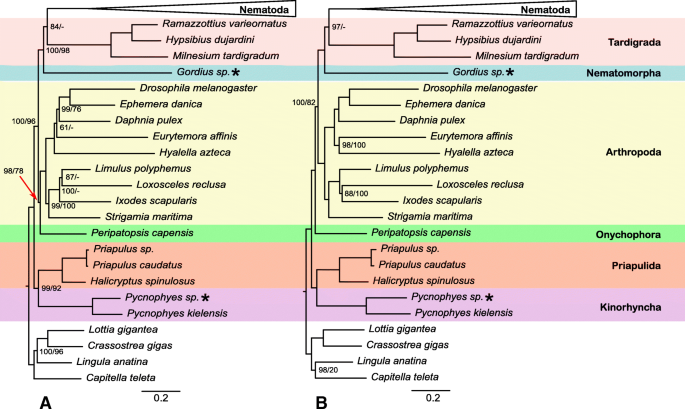
Phylogeny of outgroup taxa based on the IQ-TREE maximum likelihood analysis of the PhyloTreePruner ( a ) and SCaFoS ( b ) datasets. IQ-TREE / RAxML bootstrap support values < 100% are shown. Newly generated transcriptomes are marked with an asterisk
Deep nematode phylogeny
Early evolution and diversification of nematodes has been a matter of much controversy (reviewed by [ 4 , 15 , 21 , 22 , 54 ]). Molecular phylogenetic studies have generally supported the existence of three major lineages and the monophyly of Chromadoria, but resolution of the deepest splits within Nematoda - relationships among Enoplia, Dorylaimia, and Chromadoria - has been recalcitrant. As in prior analyses based on 18S rDNA [ 7 , 36 , 53 ], analysis of our PhyloTreePruner-based dataset lacked support for relationships among these deepest branches in Nematoda. Enoplia received moderate support (bs = 88), while monophyly of Enoplida could not be established. Insufficient taxon sampling and limited matrix occupancy for Enoplia is, in our opinion, the prime issue to be considered and addressed in efforts to resolve relationships among these deep branches.
Our initial dataset assembly strategy employed PhyloTreePruner [ 49 ], which helps exclude paralogous sequences and contamination missed by the initial orthology inference approach. PhyloTreePruner examines single-gene trees and, if there are two or more sequences from a taxon that do not form a clade, the tree is pruned to the largest subclade in which all taxa are represented by just one sequence. Only the subset of sequences corresponding to that subtree is retained for concatenation and species tree reconstruction. Unfortunately, the PhyloTreePruner algorithm can result in the unnecessary exclusion of large numbers of sequences when even a single taxon has two or more sequences that do not form a clade in single-gene trees (Thálen and Kocot, unpublished data). Aside from paralogy, putative single-gene trees with two or more sequences from the same taxon that do not form a clade may also be caused by the presence of very short and/or mis-aligned contigs, low-quality contigs, or incorrect single gene trees. This problem is exacerbated as the number of sampled taxa increases (Thálen and Kocot, unpublished data).
Use of PhyloTreePruner with its strict orthology inference approach on this rather species-rich dataset resulted in exclusion of large subtrees worth of sequences for many of the orthogroups identified by HaMStR and a final concatenated dataset with just 15.33% matrix completeness. Because the HaMStR “model organisms” core ortholog set used in this study is known to consist of genes that are single copy across diverse metazoan phyla [ 48 ], paralogy is unlikely to be problematic with this dataset (although taxon-specific gene duplications are possible). Thus, we re-ran our pipeline using SCaFoS [ 50 ] to select sequences for concatenation. SCaFoS excludes highly divergent sequences (i.e., it is still able to exclude, non-nematode contamination) and selects the best sequence for each taxon based on average p-distance. As noted above, this resulted in a larger and much more complete dataset (64.99% matrix occupancy).
Despite substantial differences in matrix completeness, analysis of the SCaFoS-based matrix resulted in a very similar topology to that of the PhyloTreePruner-based matrix. Of significance, analysis of this more complete data matrix resulted in strong support for relationships among the major lineages of Nematoda, placing a monophyletic Enoplia sister to all other nematodes with maximal support, and supporting the monophyly of Enoplida. Our SCaFoS-based phylogeny supports the “traditional” view of early nematode evolution with Enoplia sister to the rest of Nematoda, a topology used as a basis for the long-standing yet poorly explored hypothesis that the phylum arose in the marine environment [ 22 , 24 , 26 , 35 ]. The alternative hypothesis of the primarily terrestrial Dorylaimia as the sister to the rest of Nematoda [ 22 ], receives no support from either of our analyses.
Placement of Enoplia as sister to the rest of Nematoda, however, does not deny the possibility of a terrestrial origin of Nematoda [ 22 ] as early-branching clades are equally represented by marine, freshwater and terrestrial taxa (Fig. 4 ). Enoplia splits into predominantly marine Enoplida and predominantly freshwater/terrestrial Triplonchida, while its sister clade (unnamed, containing the rest of Nematoda) consists of primarily marine Chromadoria and primarily freshwater/terrestrial Dorylaimia. A comprehensive hypothesis of nematode origin and early evolution must build on a greatly expanded phylogenomic dataset with better sampling of Enoplia and Dorylaimia and closely related phyla (Nematomorpha, Tardigrada, Priapulida, Kinorhyncha and Loricifera, Onychophora). This would better enable ancestral character state reconstruction analysis for Nematoda and Ecdysozoa as a whole.
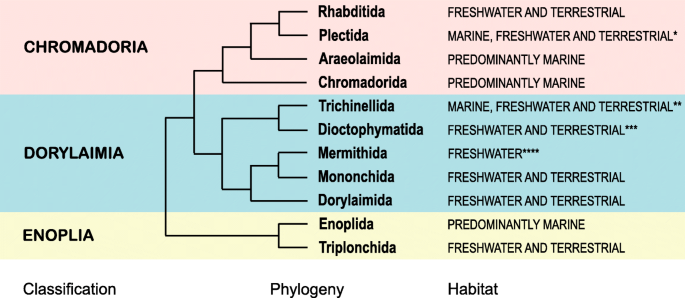
Simplified nematode phylogeny based on Fig. 2 indicating marine versus freshwater/terrestrial distribution for each order, considering the distribution of the majority of species. Notes: * includes equal number of marine, freshwater and terrestrial taxa, with molecular phylogenies suggesting terrestrial clades to be earlier (deeper); ** based on distribution of hosts, marine taxa may be of secondary origin; *** based on distribution of hosts; **** based on distribution of hosts and free-living stages
Relationships within major nematode clades
In terms of relationships within major nematode clades, our results are largely consistent with earlier studies based on the 18S rDNA gene [ 27 , 30 , 36 , 53 ] and previous phylogenomic studies [ 40 , 42 ]. One exception is the topology within Dorylaimia, which is somewhat different: 18S rDNA-based trees place Dorylaimida as the earliest branching clade [ 36 , 53 ], although relationships among Mononchida, Mermithida, Trichinellida and Dioctophymatida vary. Our results place a clade containing Dorylaimida, Mermithida and Mononchida sister to a clade with Dioctophymatida and Trichinellida. Our recovery of Mermithida as the sister taxon of Mononchida is in agreement with 18S rDNA based phylogenetic studies (e.g. [ 27 ], but in discordance with morphology-based theories, which suggest closer affinities between Mermithida and Dorylaimida [ 55 , 56 ] or Mermithida and Trichinellida (=Trichocephalida) [ 57 ]. Another exception is in the branching pattern of Rhabditida: our analysis places Spirurina as a sister to Tylenchina + Rhabditina (in full agreement with all 18S rDNA-based and most phylogenomic studies), while [ 42 ] recovered Tylenchina as a sister to Rhabditina + Spirurina, albeit with relatively low bootstrap support.
“Minor” problems in nematode phylogeny
Early radiation within the phylum Nematoda is the most challenging problem but not the only one in the systematics of this group of animals. There are a number of “orphaned” nematode taxa for which phylogenetic affinities and thus placement in the classification remain unclear. Such are the phylogenetic relationships of nematode families Teratocephalidae [ 22 ], Chambersiellidae [ 58 ], Brevibuccidae [ 22 ], Myolaimidae [ 59 ], Aegialoalaimidae [ 60 ], Cyartonematidae [ 61 ], Aulolaimidae [ 62 , 63 ], Paramicrolaimidae [ 60 , 64 ], Haliplectidae [ 60 ], Richtersiidae [ 65 ], Rhabdodemaniidae [ 51 , 66 ], Thalassogeneridae [ 67 ], suborder Ceramonematina [ 60 ] and orders Benthimermithida [ 68 , 69 ], Marimermithida [ 70 ] and Rhaptothyreida [ 71 ]. They often possess unusual morphologies [ 59 , 63 , 64 ] or are highly specialized parasites [ 69 , 70 ], and have no clear place in morphology-based classifications.
Acquisition of transcriptome or genome data from the understudied taxa is needed in order to resolve these “minor” phylogenetic issues that could not be clarified in phylogenetic studies based on rDNA loci or morphology, which have provided contradictory results depending on the data or methodology used. Besides finally achieving stable classification, many of these taxa are important for understanding of morphological character evolution, transitions between marine and terrestrial lifestyles, and evolution of symbiosis in the marine environment.
Phylogeny of Ecdysozoa
Although taxon sampling of the present study focused on Nematoda, we aimed to broadly sample relevant outgroups using only high-quality, publicly available data plus new transcriptomes from a nematomorph and a kinorhynch. Relationships among ecdysozoan phyla have varied somewhat dramatically among studies (reviewed by [ 72 ]), prompting numerous conflicting phylogenetic hypotheses. Our results find no support for some traditionally hypothesized groups including Nematoida (Nematoda + Nematomorpha), Panarthropoda (Arthropoda, Onychophora, and Tardigrada), or Cycloneuralia (Scalidophora + Nematoida). Interestingly, we recover Tardigrada as the sister taxon of Nematoda. A close relationship of Tardigrada to Nematoda has been recovered in other recent phylogenomic studies [ 73 , 74 , 75 , 76 , 77 ], but data from representatives of Nematomorpha have been limited. Interestingly, the PhyloTreePruner-based analysis recovers the traditionally hypothesized placement of Onychophora as the sister taxon of Arthropoda with strong support (bs = 98) but in the SCaFoS-based analysis, it is recovered as the sister taxon of a clade of all other non-scalidophoran ecdysozoans with maximal support. The limited taxon sampling for key ecdysozoan clades (e.g., just one onychohoran, one nematomorph, no heterotardigrades, no loriciferans, etc.) further demonstrates the need for high-quality genomic and transcriptomic resources from this part of the animal tree.
Expand sampling of free-living nematodes to learn more about parasites
The origin and evolution of animal parasitic nematodes from their free-living ancestors has been an active area of research for 80 years [ 78 , 79 , 80 , 81 , 82 , 83 ]. Two simplified scenarios describe evolutionary pre-adaptations and morpho-physiological changes leading towards parasitism via commensalism in aquatic environments [ 84 , 85 ] and via a saprobiontic lifestyle in terrestrial environments [ 80 , 86 , 87 ]. We are just beginning to understand the genomic changes involved in these processes [ 42 , 88 ]. Furthermore, many other important questions about parasite biology remain unanswered, such as how parasites locate and invade hosts, suppress host immune response, acquire nutrients, etc. [ 40 ].
Comprehensive understanding of morphological, ecological, behavioral and genomic adaptations involved in the evolution of a parasitic lifestyle can not be achieved without thorough comparison between parasites and their close, free-living relatives [ 19 , 40 , 89 ]. One of the complications, however, is that animal parasitic nematodes evolved independently at least 18 times [ 90 ], if not more [ 40 , 86 ], and one cannot expect the same underlying mechanism to be behind these numerous independent events. Moreover, the majority of animal parasitic clades have no identified, closely related free-living taxon suitable for comparative analysis [ 19 ]. These include all parasites from the subclass Dorylaimia and the most diverse and economically important Spirurina. Even the closest relative of such a well-researched taxon as the entomopathogenic genus Steinernema remains unclear [ 58 , 91 ]. Thus, further expanding sampling of free-living nematodes in phylogenomic studies will be an integral part of any future research aiming to understand the evolution of parasitism – it will help elucidate sister-group relationships of those parasitic taxa for which the closest free-living relatives are yet unidentified and provide much needed comparative data for identification of parasitism-related genetic modifications.
With 97 published and nine new nematode genomes and transcriptomes, our phylogenetic analyses, which are by far the most comprehensive to date, cover less than 0.5% of the approximately 23,000 valid nematode species [ 92 ]. For comparison, the latest phylum-wide 18S rDNA-based phylogeny [ 53 ] included 1215 sequences or just about 5% of the known diversity. Of the 108 nematode species included in our analyses, 80 belong to Rhabditida – a clade with over 13,400 known species including most economically and medically important parasites as well as the model species Caenorhabditis elegans and satellite model Pristionchus pacificus . Of the Rhabditida species included in our analyses, 50 are parasites of animals, 12 are plant parasitic, and the remaining 18 are thought to be free-living inhabitants of soil or saprophytic communities (although some are phoretically associated with invertebrates). The next largest set of species, 11 in number, represent exclusively the parasitic order Trichinellida (with about 400 known species). The remainder of the phylum, consisting of 17 orders and including free-living (in particular almost all known marine species), plant- and animal parasitic nematodes (with about 9200 species in total), is unevenly represented in our analysis: nine orders are represented by 17 species, while eight orders are not included at all. Out of 108 species included in this phylogenetic analysis, 63 are animal parasitic and 14 are associated with plants, while only 31 are free-living, of which 22 are fresh water and soil inhabitants and only nine are marine. Thus, vast habitat diversity, and the morphological and molecular adaptations that allow nematodes to live in those environments, remains unrepresented in transcriptome-based phylogenies.
Recommended sampling strategies
Three possible sampling strategies to increase and diversify nematode genomic and transcriptomic datasets can be suggested, depending on the research goals. Those researchers who are interested solely in the origin and early evolution of animal parasitism can find interesting models among free-living Enoplida [ 93 ], Chromadorida [ 94 ], Monhysterida [ 95 , 96 ] and Plectida [ 97 , 98 , 99 ] – species with parasitic lifestyles but with morphology retaining many features of their close, free-living relatives. Phylogenetic analysis and subsequent ancestral character state reconstruction would elucidate features of free-living ancestors of parasites and generate new hypotheses regarding the evolution of parasitism. Secondly, studies aimed at improving general nematode phylogeny and classification must focus on the species described above in the “Minor” problems in nematode phylogeny and taxa to which they were once believed to be related to. Finally, large taxonomic categories currently represented by single or few genomes/transcriptomes (Triplonchida, Mononchida, Dorylaimida, etc) also deserve attention, and further sampling of those taxa would elucidate relationships in those clades and likely spur research into yet more unanswered questions.
This study represents the largest phylogenomic analysis of nematodes to date, and furthers our understanding of nematode relationships. We have also, however, revealed how poorly sampled the current dataset is relative to the tremendous diversity of nematodes on Earth. Sequencing and re-sequencing of more species and broad scale comparative studies can also reveal and correct misidentified or mislabelled datasets (the case of Heterorhabditis indica ). Transcriptome sequencing of nematodes is still strongly biased toward parasitic and “model” taxa, particularly those in the Rhabditida, neglecting the free-living clades that hold the key to the origins of the phylum. Our understanding of nematode early evolution and various pathways towards parasitism will be improved only by broader sampling and sequencing of free-living taxa.
Nematodes and the kinorhynch were collected and isolated following standard protocols for sampling meiofauna [ 100 ]. Immediately after isolation, live specimens of Anaplectus granulosus, Euchromadora sp., Symplocostoma sp., and Tobrilus sp. were frozen in 100 μL of nuclease-free water at − 70 °C. Bathylaimus sp., Gordius sp., Odontophora sp., Oncholaimidae sp., Pontonema sp., Pycnophyes sp., and Thoracostomopsidae sp. were preserved in RNA later and stored at − 20 °C.
Total RNA was extracted from all samples but Gordius sp. using the Ambion RNAqueous-Micro Kit. For Anaplectus granulosus, Euchromadora sp. and Tobrilus sp., 1000 μL of lysis solution was added directly to the original sample (nematodes in 100 μl of nuclease-free water), while individual specimens of the remaining nematodes and the kinorhynch were manually transferred from RNAlater or nuclease-free water to lysis solution. Subsequent steps of RNA extraction and DNAse treatment followed the manufacturer’s protocol. RNA was extracted from the nematomorph Gordius sp. using the Omega Bio-Tek EZNA Mollusc RNA kit using a rotor-stator homogenizer for homogenization and on-column DNAse treatment.
For Anaplectus granulosus , Bathylaimus sp., Euchromadora sp., Odontophora sp., Pontonema sp., Symplocostoma sp. and Tobrilus sp., library preparation and cDNA synthesis was performed using the Clontech SMARTer PCR cDNA Synthesis Kit following manufacturer’s instructions. Resulting double-stranded cDNA was purified using the QIAquick PCR Purification Kit. Concentration of double-stranded cDNA was measured using Qubit dsDNA HS Assay Kit and Qubit 3.0 Fluorometer. Final library preparation and transcriptome sequencing were performed at the Swedish National Genomics Infrastructure in Stockholm, Sweden using the Illumina TruSeq PCR-free protocol and an Illumina HiSeq 2500 in high-output mode with V4 2 X 125 bp paired-end reads.
For Oncholaimidae sp. and Thoracostomopsidae sp., total RNA (not quantified; < 1 ng) was sent to Macrogen Inc. (Seoul, South Korea) for cDNA library preparation with the SMARTer low input RNA kit and sequencing using on the Illumina HiSeq 2500 using HiSeq SBS V4 with 2 X 100 bp paired-end reads. For Gordius sp., total RNA (1 μg) was sent to Macrogen for Illumina TruSeq RNA library preparation and sequencing using the Illumina HiSeq 2500 using HiSeq SBS V4 with 2 X 100 bp paired-end reads.
Dataset assembly and analysis followed the approach of Kocot et al. [ 47 ]. Publicly available genomic data [ 101 , 102 ] were downloaded as predicted proteins if available (Additional file 2 : Table S1). Transcriptome dataset of Plectus sambesii was provided by Dr. Philipp Schiffer (CLOE, University College London, UK) and Dr. Christopher Kraus (Zoological Institute, Universität zu Köln, Germany), while transcriptome of Pontonema vulgare was provided by Dr. Andreas Hejnol (Sars International Centre for Marine Molecular Biology, University of Bergen, Norway). Otherwise, predicted transcripts from genomes or assembled transcriptomes were downloaded when possible. After demultiplexing, raw reads for Anaplectus granulosus , Bathylaimus sp., Euchromadora sp., Odontophora sp., Pontonema sp., Symplocostoma sp. and Tobrilus sp. were filtered using AfterQC [ 103 ] and assembled with Trinity [ 104 ] installations available on public Galaxy [ 105 ] servers at usegalaxy.org (Center for Comparative Genomics and Bioinformatics at Penn State, the Department of Biology and at Johns Hopkins University and the Computational Biology Program at Oregon Health & Science University) or galaxy.ncgas-trinity.indiana.edu (National Center for Genome Analysis Support, Pervasive Technology Institute at Indiana University). Pycnophyes sp., Gordius sp., Oncholaimidae sp. and Thoracostomopsidae sp. as well as publicly available transcriptomes available only as raw reads were quality filtered, adapter-trimmed, and assembled using Trinity 2.2.0 with the --trimmomatic and --normalize_reads flags [ 104 ] on the University of Alabama UAHPC cluster. Transcripts were translated with TransDecoder 2.0.0 or 2.0.1 [ 106 ] using the UniProt SwissProt database (accessed on September 20th, 2016; The Uniprot Consortium 2014) and PFAM (Pfam-A.hmm) version 27 [ 107 ].
For orthology inference, HaMStR 13 [ 48 ] was used with the “model organisms” core-ortholog set. Translated transcripts for all taxa except Caenorhabditis elegans were searched against the 1031 profile hidden Markov models (pHMMs) using the “-central” flag and otherwise with the default options. Sequences matching a pHMM were compared to the proteome of Caenorhabditis elegans using BLASTP with the default search settings of HaMStR. If the Caenorhabditis elegans amino acid sequence contributing to the pHMM was the best BLASTP hit in each of these back-BLASTs, the sequence was then assigned to that putative orthology group (simply referred to as “gene” henceforth). Redundant sequences that were identical (including partial sequences that were identical at least where they overlapped) were then removed with UniqHaplo ( http://raven.wrrb.uaf.edu/~ntakebay/teaching/programming/perl-scripts/uniqHaplo.pl ), leaving only unique sequences for each taxon. Each gene was then aligned with MAFFT 7.215 using the automatic alignment strategy with a “maxiterate” value of 1000 [ 108 ]. Alignments were then trimmed with BMGE (−g 0.5) to remove ambiguously aligned regions and any alignments shorter than 50 bp were deleted. Sequences that did not overlap with all other sequences in the alignment by at least 20 amino acids were deleted, starting with the shortest sequences not meeting this criterion. This step was necessary for downstream single-gene tree reconstruction. Finally, genes sampled for fewer than 10 taxa after these steps were discarded.
In some cases, a taxon was represented in an alignment by two or more sequences (splice variants, lineage-specific gene duplications [=inparalogs], undetected paralogs, or exogenous contamination). To screen for evidence of paralogy or contamination and select just one sequence for each taxon, an approximately maximum likelihood tree was inferred for each remaining alignment using FastTree 2 [ 109 ] using the -slow and -gamma options. PhyloTreePruner [ 49 ] was then employed to use a tree-based approach to screen each single-gene alignment for evidence of paralogy or contamination. First, nodes with support values below 0.95 were collapsed into polytomies. Next, the maximally inclusive subtree was selected where each taxon was represented by no more than one sequence or, in cases where more than one sequence was present for any taxon, all sequences from that taxon formed a clade or were part of the same polytomy. Putative paralogs and contaminants (sequences falling outside of this maximally inclusive subtree) were then deleted from the input alignment. In cases where multiple sequences from the same taxon formed a clade or were part of the same polytomy, all sequences except the longest were deleted. Concatenation of remaining sequences to assemble the data matrix henceforth referred to as the “original full dataset” was performed using FASconCAT-G [ 110 ].
Because PhyloTreePruner can result in the unnecessary exclusion of large numbers of sequences when even a single taxon has unstable or contaminant sequences, we also ran our pipeline using SCaFoS [ 50 ] instead of PhyloTreePruner. The default settings were used to exclude highly divergent sequences and select the best sequence for each taxon based on average p-distance to all other sequences in the alignment.
Maximum likelihood (ML) analyses were conducted in RAxML 8.2.8 [ 111 ] and IQ-TREE [ 112 ]. Because of the very large number of taxa in our matrices, for the RAxML analyses, data matrices were partitioned by gene but the PROTGAMMALG model was specified for all partitions rather than empirically inferring the best-fitting model for each partition. A preliminary run of PartitionFinder 2 [ 113 ] found that the LG model was the best fit for the vast majority of partitions. The tree with the best likelihood score after 10 random addition sequence replicates was retained and nodal support was assessed with rapid bootstrapping with the number of replicates determined by the autoMRE criterion. IQ-TREE analyses were performed using IQ-TREE 1.5.5 with the site heterogeneous PMSF model [ 63 ] (−m LG + C20 + G + F) with the RAxML bipartitions tree provided as the required guide tree (−ft). Nodal support was assessed with 1000 rapid bootstraps (−bb 1000).
Several taxonomy assignment approaches were used to identify the transcriptome of Heterorhabditis indica . At first, transcriptome database for Heterorhabditis indica available at http://insilico.iari.res.in/hindica/ was mined for possible ribosomal DNA sequences using built-in BLAST search and 18S rDNA sequence of Plectus aquatilis (chosen to be equally distantly related from both Heterorhabditis and Heterodera ) as a target. Four recovered transcripts were then compared with the publicly available sequences from the nucleotide collection of NCBI GenBank using blastn suite (alignment-based taxonomy assignment approach, see review in [ 114 ]). One of the recovered transcripts (labelled as Locus_123_Transcript_1/1) showed high similarity (> 99% identity, E-value = 0) to several 18S sequences from different species of the genera Heterodera and Globodera , with Heterodera glycines (GenBank acc. Number AY043247) having the highest identity score, albeit with partial overlap. The other two transcripts (labelled as Locus_90_Transcript_1/2 and Locus_90_Transcript_2/2 respectively) also showed high similarity (99% identity, E-value = 0) to several sequences from different species of the genera Heterodera and Globodera , partially overlapping various reference sequences that may include partial 18S, ITS1, 5.8S, ITS2 and partial 28S, with Heterodera cajani (GenBank acc. Number AY274389) having the highest identity score. Similar results were obtained by mining the transcriptome assembly downloaded directly from GenBank.
The longest section of 18S rDNA sequence mined from the Heterorhabditis indica transcriptome database (588 base long partial 5′ section from the Locus_123_Transcript_1/1) was then used in tree-based taxonomy assignment approach (see review in [ 115 ]) to double-check the results of alignment-based taxonomy assignment. This section was added to a selection of 18S rDNA sequences downloaded from SILVA database [ 116 ] and representing all major clades of Rhabditida, including all available near-full length sequences for identified species from the genera Heterorhabditis, Heterodera and Globodera. The alignment was created using MUSCLE at https://www.ebi.ac.uk/Tools/msa/muscle/ under default settings and trimmed to a size of a fragment from the Heterorhabditis indica transcriptome. A phylogenetic tree was inferred using RAxML-HPC2 under default settings with 1000 bootstrap replicates.
Availability of data and materials
The datasets generated and analysed during the current study including transcriptome assemblies are available via FigShare: https://figshare.com/s/4c8e501714dbd5be1be8
Abbreviations
Bootstrap support
Maximum likelihood
Hugot JP, Baujard P, Morand S. Biodiversity in helminths and nematodes as a field of study: an overview. Nematology. 2001;3(3):199–208.
Article Google Scholar
Baldwin JG, Nadler SA, Wall DH. Nematodes: pervading the earth and linking all life. In: Raven P, Williams T, editors. Nature and human society: the quest for a sustainable world: proceedings of the 1997 forum on biodiversity, vol. 2000. Washington DC: National Academy Press; 2000. p. 176–91.
Google Scholar
Appeltans W, Ahyong ST, Anderson G, Angel MV, Artois T, Bailly N, et al. The magnitude of global marine species diversity. Curr Biol. 2012;22(23):2189–202.
Article CAS PubMed Google Scholar
Blaxter M, Koutsovoulos G, Jones M, Kumar S, Elsworth B. Phylogenomics of Nematoda. In: Cotton JA, Hughes J, Olson P, editors. Next-generation systematics. Cambridge: Cambridge University Press; 2016. p. 62–83. https://books.google.com/books?hl=en&lr=&id=C3JNDAAAQBAJ&oi=fnd&pg=PR9&dq=Blaxter+M,+Koutsovoulos+G,+Jones+M,+Kumar+S,+Elsworth+B.+Phylogenomics+of+Nematoda.+In:+Cotton+JA,+Hughes+J,+Olson+P,+editors.+Nextgeneration+systematics:+Cambridge+University+Press%3B+2016.+p.+62%E2%80%9383.&ots=a4ebTDtW03&sig=J4P4jtLKs0kRpj-B_MiyGBwpdOQ#v=onepage&q&f=false .
Warwick RM, Rice R. Ecological and metabolic studies on free living nematodes from an estuarine mudflat. Estuar Coast Shelf Sci. 1979;9:251–7.
Lambshead PJD, Schalk P. Overview of marine invertebrate biodiversity. In: Levin S, editor. Encyclopedia of biodiversity, vol. 3. Cambridge, MA: academic press; 2001. p. 543–9.
Chapter Google Scholar
Bik HM, Lambshead PJD, Thomas WK, Lunt DH. Moving towards a complete molecular framework of the Nematoda: a focus on the Enoplida and early-branching clades. BMC Evol Biol. 2010;10:353.
Article PubMed PubMed Central Google Scholar
Fonseca VG, Carvalho GR, Sung W, Johnson HF, Power DM, Neill SP, et al. Second-generation environmental sequencing unmasks marine metazoan biodiversity. Nat Commun. 2010;1:98.
Article PubMed CAS Google Scholar
Coull BC. Are members of the meiofauna food for higher trophic levels? Trans Am Microsc Soc. 1990;109:233–46.
Lambshead P, Brown C, Ferrero T, Hawkins L, Smith C, Mitchell N. Biodiversity of nematode assemblages from the region of the clarion-Clipperton fracture zone, an area of commercial mining interest. BMC Ecol. 2003;3(1):1–12.
Bert W, Manhout J, Van Colen C, Borgonie G, Decraemer W. Nematode assemblages in a nature reserve with historical pollution. Belg J Zool. 2009;139:3–14.
Gingold R, Moens T, Rocha-Olivares A. Assessing the response of nematode communities to climate change-driven warming: a microcosm experiment. PLoS One. 2013;8(6):e66653.
Article CAS PubMed PubMed Central Google Scholar
Andrus P, Rae R. Development of Phasmarhabditis hermaphrodita (and members of the Phasmarhabditis genus) as new genetic model nematodes to study the genetic basis of parasitism. J Helminthol. 2018;93(3):319-31. https://www.ncbi.nlm.nih.gov/pubmed/29607798 .
Derycke S, Backeljau T, Moens T. Dispersal and gene flow in free-living marine nematodes. Front Zool. 2013;10(1):1.
Chitwood BG, Chitwood MB. Introduction to nematology, revised edition. Baltimore: University Park Press; 1974.
Maggenti A. General Nematology. New York: Springer-Verlag; 1981.
Book Google Scholar
De Ley P. A quick tour of nematode diversity and the backbone of nematode phylogeny. In The C. elegans Research Community, editor. WormBook. (January 25, 2006). https://doi.org/10.1895/wormbook.1.41.1 .
Copley JTP, Flint HC, Ferrero TJ, Van Dover CL. Diversity of melofauna and free-living nematodes in hydrothermal vent mussel beds on the northern and southern east pacific rise. J Mar Biol Assoc UK. 2007;87:1141–52.
Viney M. How can we understand the genomic basis of nematode parasitism? Trends Parasitol. 2017;33(6):444–52.
Dorris M, De Ley P, Blaxter M. Molecular analysis of nematode diversity and the evolution of parasitism. Parasitol Today. 1999;15(5):188–93.
De Ley P, Blaxter ML. Systematic position and phylogeny. In: Lee D, editor. The biology of nematodes. London: Taylor and Francis; 2002. p. 1–30.
De Ley P, Blaxter ML. A new system for Nematoda: combining morphological characters with molecular trees, and translating clades into ranks and taxa. Nematology Monographs and Perspectives. 2004;2:633–53.
Chitwood BG. The designation of official names for higher taxa of invertebrates. Bull Zool Nomencl. 1958;15:860–95.
Maggenti AR. Comparative morphology in nemic phylogeny. In: Dougherty E, Brown Z, Hanson E, Hartman W, editors. The lower Metazoa, comparative biology and phylogeny. Berkeley: University of California Press; 1963. p. 273–82.
Andrássy I. Evolution as a basis for the systematization of nematodes. London: Pitman Publishers; 1976.
Malakhov VV. Nematodes: structure, development, classification, and phylogeny. Washington, DC: Smithsonian Institution Press; 1994.
Blaxter ML, De Ley P, Garey JR, Liu LX, Scheldeman P, Vierstraete A, et al. A molecular evolutionary framework for the phylum Nematoda. Nature. 1998;392(6671):71–5.
Smythe A, Sanderson M, Nadler S. Nematode small subunit phylogeny correlates with alignment parameters. Syst Biol. 2006;55(6):972–92.
Article PubMed Google Scholar
Donn S, Neilson R, Griffiths BS, Daniell TJ. Greater coverage of the phylum Nematoda in SSU rDNA studies. Biol Fertil Soils. 2011;47:333.
Article CAS Google Scholar
Holterman M, van der Wurff A, van den Elsen S, van Megen H, Bongers T, Holovachov O, et al. Phylum-wide analysis of SSU rDNA reveals deep phylogenetic relationships among nematodes and accelerated evolution towards crown clades. Mol Biol Evol. 2006;23:1792–800.
Hope I. Embryology, developmental biology and the genome. In: Lee D, editor. The biology of nematodes. London: Taylor and Francis; 2002. p. 121–45.
Schierenberg E. Unusual cleavage and gastrulation in a freshwater nematode: developmental and phylogenetic implications. Dev Genes Evol. 2005;215(2):103–8.
Schulze J, Schierenberg E. Evolution of embryonic development in nematodes. EvoDevo. 2011;2:18.
Justine J. Male and female gametes and fertilisation. In: Lee D, editor. The biology of nematodes. London: Taylor and Francis; 2002. p. 73–119.
Paramonov AA. Principles of phytonematology Vol. 1. The origin of nematodes. Ecological and morphological characteristics of phytonematodes. General principles of taxonomy. Izdatelstvo Akademii Nauk SSSR; 1964.
Meldal BHM, Debenham NJ, De Ley P, De Ley IT, Vanfleteren JR, Vierstraete A, et al. An improved molecular phylogeny of the Nematoda with special emphasis on marine taxa. Mol Phylogenet Evol. 2007;42:622–36.
Parkinson J, Mitreva M, Whitton C, Thomson M, Daub J, Martin J, et al. A transcriptomic analysis of the phylum Nematoda. Nat Genet. 2004;36(12):1259–67.
Wasmuth J, Schmid R, Hedley A, Blaxter M. On the extent and origins of genic novelty in the phylum Nematoda. PLoS Negl Trop Dis. 2008;2(7):e258.
Article PubMed PubMed Central CAS Google Scholar
Blaxter M, Kumar S, Kaur G, Koutsovoulos G, Elsworth B. Genomics and transcriptomics across the diversity of the Nematoda. Parasite Immunol. 2012;34(2–3):108–20.
Blaxter M, Koutsovoulos G. The evolution of parasitism in Nematoda. Parasitology. 2015;142(Suppl 1):S26–39.
Koutsovoulos G. Reconstructing the phylogenetic relationships of nematodes using draft genomes and transcriptomes. PhD [dissertation]. Edinburgh, Scotland: University of Edinburgh; 2015.
International Helminth Genomes Consortium. Comparative genomics of the major parasitic worms. Nat Genet. 2018. https://doi.org/10.1038/s41588-018-0262-1 .
Kang S, Sultana T, Eom KS, Park YC, Soonthornpong N, Nadler SA, et al. The mitochondrial genome sequence of Enterobius vermicularis (Nematoda: Oxyurida) – an idiosyncratic gene order and phylogenetic information for chromadorean nematodes. Gene. 2009;429:87–97.
Kim J, Lee S-H, Gazi M, Kim T, Jung D, Chun J-Y, et al. Mitochondrial genomes advance phylogenetic hypotheses for Tylenchina (Nematoda: Chromadorea). Zool Scr. 2015;44(4):446–62.
Kim J, Kern E, Kim T, Sim M, Kim J, Kim Y, et al. Phylogenetic analysis of two Plectus mitochondrial genomes (Nematoda: Plectida) supports a sister group relationship between Plectida and Rhabditida within Chromadorea. Mol Phylogenet Evol. 2017;107:90–102.
Park J-K, Sultana T, Lee S-H, Kang S, Kim HK, Min G-S, et al. Monophyly of clade III nematodes is not supported by phylogenetic analysis of complete mitochondrial genome sequences. BMC Genomics. 2011;12:392.
Kocot KM, Tassia MG, Halanych KM, Swalla BJ. Phylogenomics offers resolution of major tunicate relationships. Mol Phylogenet Evol. 2018;121:166–73.
Ebersberger I, Strauss S, vo Haeseler A. HaMStR: profile hidden markov model based search for orthologs in ESTs. BMC Evol Biol. 2009;9:157.
Kocot KM, Citarella MR, Moroz LL, Halanych KM. PhyloTreePruner: a phylogenetic tree-based approach for selection of orthologous sequences for Phylogenomics. Evol Bioinformatics Online. 2013;9:429–35.
CAS Google Scholar
Roure B, Rodriguez-Ezpeleta N, Philippe H. SCaFoS: a tool for selection, concatenation and fusion of sequences for phylogenomics. BMC Evol Biol. 2007;7(Suppl. 1):S2.
Smythe AB. Evolution of feeding structures in the marine nematode order Enoplida. Integr Comp Biol. 2015;55(2):228–40.
Somvanshi VS, Gahoi S, Banakar P, Thakur PK, Kumar M, et al. A transcriptomic insight into the infective juvenile stage of the insect parasitic nematode, Heterorhabditis indica . BMC Genomics. 2016;17:166.
van Megen H, van den Elsen S, Holterman M, Karssen G, Mooyman P, Bongers T, et al. A phylogenetic tree of nematodes based on about 1200 full-length small subunit ribosomal DNA sequences. Nematology. 2009;11:927–50.
Coomans A. Present status and future of nematode systematics. Nematology. 2002;4(5):573–82.
Steiner G. Über die Verwandtschaftsverhältnisse und die systematische Stellung der Mermithiden. Zool Anz. 1917;48(9):263–7.
Poinar GO Jr, Hess R. Structure of the pre-parasitic juveniles of Filipjevimermis leipsandra and some other Mermithidae (Nematodea). Nematologica. 1974;20:163–73.
Rubtsov IA. Mermithids. Origin, biology, distribution. Nauka; 1977.
Holovachov O, Camp L, Nadler SA. Sensitivity of ribosomal RNA character sampling in the phylogeny of Rhabditida. J Nematol. 2015;47:337–55.
CAS PubMed PubMed Central Google Scholar
Slos D, Couvreur M, Bert W. Description of Myolaimus mycophilus Slos & Bert sp. n. (Rhabditida: Myolaimidae). Nematology. 2018;20(2):175–86.
Holovachov O. Chapter 7.16: order Plectida Gadea, 1973. In: Schmidt-Raesa A, editor. Handbook of zoology. Gastrotricha, Cycloneuralia and Gnathifera. Volume 2: Nematoda. Hamburg: De Gruyter; 2014. p. 487–536.
Tchesunov AV. On the morphology and systematic position of the family Meyliidae (Nematoda: Chromadoria). Nematologica. 1994;40:369–78.
Holovachov O, Bostrőm S, Susulovsky A. Description of Aulolaimus multipapillatus sp. n. and A. nannocephalus Andrássy, 1972 with notes on taxonomy and phylogeny of the genus (Nematoda: Aulolaimidae). Nematology. 2007;9(2):201–14.
Abolafia J, Peña-Santiago R. Morphology, taxonomy and phylogeny of the enigmatic genus Aulolaimus de man, 1880 (Nematoda, Aulolaimidae). Zool Anz. 2018;277:101–15.
Leduc D, Verdon V, Zhao Z. Phylogenetic position of the Paramicrolaimidae, description of a new Paramicrolaimus species and erection of a new order to accommodate the Microlaimoidea (Nematoda: Chromadorea). Zool J Linnean Soc. 2018;183(1):52–69.
Tchesunov AV. Chapter 7.13: Order Desmodorida De Coninck, 1965. In: Schmidt-Raesa A, editor. Handbook of zoology. Gastrotricha, Cycloneuralia and Gnathifera. Volume 2: Nematoda. Hamburg: De Gruyter; 2014. p. 399–434.
Holovachov O, Shoshin A. Chapter 7.4: order Triplonchida cobb, 1919. In: Schmidt-Raesa A, editor. Handbook of zoology. Gastrotricha, Cycloneuralia and Gnathifera. Volume 2: Nematoda. Hamburg: De Gruyter; 2014. p. 251–76.
Smol N, Muthumbi A, Sharma J. Chapter 7.3: Order Enoplida. In: Schmidt-Raesa A, editor. Handbook of zoology. Gastrotricha, Cycloneuralia and Gnathifera. Volume 2: Nematoda. Hamburg: De Gruyter; 2014. p. 193–250.
Holovachov O, Rodrigues CF, Zbinden M, Duperron S. Trophomera conchicola sp. n. (Nematoda: Benthimermithidae) from chemosymbiotic bivalves Idas modiolaeiformis and Lucionoma kazani (Mollusca: Mytilidae and Lucinidae) in eastern Mediterranean. Russ J Nematol. 2013;21:1–12.
Miljutin DM. Chapter 7.1: order Benthimermithida Tchesunov, 1995. In: Schmidt-Raesa A, editor. Handbook of zoology. Gastrotricha, Cycloneuralia and Gnathifera. Volume 2: Nematoda. Hamburg: De Gruyter; 2014. p. 179–86.
Miljutin DM. Chapter 7.10: order Marimermithida Rubtzov 1980, emend. Tchesunov, 1995. In: Schmidt-Raesa A, editor. Handbook of zoology. Gastrotricha, Cycloneuralia and Gnathifera. Volume 2: Nematoda. Hamburg: De Gruyter; 2014. p. 345–50.
Leduc D, Zhao Z, Verdon V, Xu Y. Phylogenetic position of the enigmatic deep-sea nematode order Rhaptothyreida: a molecular analysis. Mol Phyl Evol. 2018;122:29–36.
Giribet G, Edgecombe GD. Current understanding of Ecdysozoa and its internal phylogenetic relationships. Integr Comp Biol. 2017;57(3):455–66.
Dunn CW, Hejnol A, Matus DQ, Pang K, Browne WE, Smith SA, Seaver E, Rouse GW, Obst M, Edgecombe GD, Sørensen MV. Broad phylogenomic sampling improves resolution of the animal tree of life. Nature. 2008;452(7188):745.
Hejnol A, Obst M, Stamatakis A, Ott M, Rouse GW, Edgecombe GD, Martinez P, Baguñà J, Bailly X, Jondelius U, Wiens M. Assessing the root of bilaterian animals with scalable phylogenomic methods. Proc R Soc London B: Biol Sci. 2009;276(1677):4261–70.
Borner J, Rehm P, Schill RO, Ebersberger I, Burmester T. A transcriptome approach to ecdysozoan phylogeny. Mol Phylogenet Evol. 2014;80:79–87.
Laumer CE, Bekkouche N, Kerbl A, Goetz F, Neves RC, Sørensen MV, Kristensen RM, Hejnol A, Dunn CW, Giribet G, Worsaae K. Spiralian phylogeny informs the evolution of microscopic lineages. Curr Biol. 2015;15(3):2000–6.
Yoshida Y, Koutsovoulos G, Laetsch DR, Stevens L, Kumar S, Horikawa DD, Ishino K, Komine S, Kunieda T, Tomita M, Blaxter M. Comparative genomics of the tardigrades Hypsibius dujardini and Ramazzottius varieornatus . PLoS Biol. 2017;15(7):e2002266.
Baylis HA. Helminths and evolution. In: de Beer GR, editor. Evolution. Oxford: Clarenden Press; 1938. p. 249–70.
Dougherty EC. Evolution of zooparasitic groups in the phylum Nematoda, with special reference to host-distribution. J Parasitol. 1951;37:353–98.
Osche G. Die Präadaptation freilebender Nematoden an den Parasitismus. Zool Anz. 1956;19(Suppl):391–6.
Anderson RC. The origins of zooparasitic nematodes. Can J Zool. 1984;62:317–28.
Clark WC. Origins of the parasitic habit in the Nematoda. Int J Parasitol. 1994;24(8):1117–29.
Blaxter M. Nematoda: genes, genomes and the evolution of parasitism. Adv Parasitol. 2003;54:101–95.
Sudhaus W. Nematoden (inbesondere Rhabditiden) des Strandanwurfs und ihre Beziehungen zu Krebsen. Faun.-ökol. Mitt. 1974;4:365–400.
Tchesunov AV. Diversity and forms of evolution of the nematodes associated with marine benthic invertebrates. Uchenye Zapiski Kazanskogo gosudarstvennogo universiteta, Estestvennye nauki. 2009;151:1–17.
Sudhaus W. Evolution of insect parasitism in rhabditid and diplogastrid nematodes. In: Makarov SE, Dmitrijevic RN, editors. Advances in arachnology and developmental biology. Belgrade: SASA; 2008. p. 143–61. https://pdfs.semanticscholar.org/38df/94d708117d8fa4ace6db9ebd4d80fd5f8602.pdf .
Sudhaus W. Preadaptive plateau in Rhabditida (Nematoda) allowed the repeated evolution of zooparasites, with an outlook on evolution of life cycles within Spiroascarida. Palaeodiversity. 2010;3(Suppl):117–30.
Zarowiecki M, Berriman M. What helminth genomes have taught us about parasite evolution. Parasitol. 2015;142:S85–97.
Viney M. The genomic basis of nematode parasitism. Brief Funct Genomics. 2018;17(1):8–14.
Weinstein SB, Kuris AM. Independent origins of parasitism in Animalia. Biol Lett. 2016;12(7):20160324.
Spiridonov SE, Subbotin SA. Phylogeny and phylogeography of Heterorhabditis and Steinernema . Nematology Monographs & Perspectives. 2016;12:413–27.
Schmidt-Rhaesa A. Handbook of zoology. Gastrotricha, Cycloneuralia and Gnathifera. Volume 2: Nematoda: Walter de Gruyter GmbH; 2014. https://www.degruyter.com/view/product/180464 .
Poinar G Jr, Lewis SC, Hagen NT, Hyman B. Systematic affinity of the sea urchin parasite, Echonomermella matsi Jones & Hagen (Enoplida, Echonomermellidae). Nematology. 2011;13:747–53.
Platonova TA, Potin VV. On new genera Harpagonchus and Harpagonchoides (Nematoda, Chromadorida, Harpagonchidae fam. N.) living on the parapodia and gills of the antarctic polychaetes Aglaophamus Kinberg and Hemipodus Quatrefages. Issledovania fauny morjei. 1972;11:81–87.
Hopper BE. Theristus polychaetophilus n. sp. (Nematoda), an external parasite of the spionid polychaete Scolelepis (Scolelepis) squamata (Müller, 1806). Can J Zool. 1966;44:787–91.
Poinar G, Duarte D, Santos MJ. Halomonhystera parasitica n. sp. (Nematoda: Monhysteridae), a parasite of Talorchestia brito (Crustacea: Talitridae) in Portugal. Syst Parasitol. 2010;75:53–8.
Ivanova ES, Spiridonov SE. Two new species of creagrocercid nematodes parasitic in earthworms, with comments on the phylogenetic affiliations of the Creagrocercidae Baylis, 1943. Syst Parasitol. 2011;78:81–94.
Hope WD, Tchesunov AV. Smithsoninema inaequale n. g, and n. sp. (Nematoda, Leptolaimidae) inhabiting the test of a foraminiferan. Invert Biol. 1999;118:95–108.
Holovachov O, Boström S. Swedish Plectida (Nematoda). Part 6. Neocamacolaimus parasiticus gen. N., sp. n. Zootaxa. 2014;3821:538–50.
Higgins RP, Thiel H. Introduction to the study of meiofauna. Washington D.C.: Smithsonian Institution Press; 1988. http://www.vliz.be/en/imis?module=ref&refid=34444&printversion=1&dropIMIStitle=1 .
Lee RYN, Howe KL, Harris TW, Arnaboldi V, Cain S, Chan J, et al. WormBase 2017: molting into a new stage. Nucleic Acids Res. 2017;46(D1):D869–74.
Article PubMed Central CAS Google Scholar
Martin J, Rosa BA, Ozersky P, Hallsworth-Pepin K, Zhang X, Bhonagiri-Palsikar V, et al. Helminth.net : expansions to Nematode.net and an introduction to Trematode.net . Nuc Acids Res. 2014. https://doi.org/10.1093/nar/gku1128 .
Chen S, Huang T, Zhou Y, Han Y, Xu M, Gu J. AfterQC: automatic filtering, trimming, error removing and quality control for fastq data. BMC Bioinformatics. 2017;18(Suppl 3):80.
Grabherr MG, Haas BJ, Yassour M, Levin JZ, Thompson DA, Amit I, et al. Full-length transcriptome assembly from RNA-seq data without a reference genome. Nat Biotechnol. 2011;29(7):644–52.
Afgan E, Baker D, van den Beek M, Blankenberg D, Bouvier D, Čech M, et al. The galaxy platform for accessible, reproducible and collaborative biomedical analyses: 2016 update. Nucleic Acids Res. 2016;44(W1):W3–W10.
Haas BJ, Papanicolaou A, Yassour M, et al. De novo transcript sequence reconstruction from RNA-seq using the trinity platform for reference generation and analysis. Nat Protoc. 2013;8(8):1494–512.
Finn RD, Coggill P, Eberhardt RY, Eddy SR, Mistry J, Mitchell AL, et al. The Pfam protein families database: towards a more sustainable future. Nuc Acids Res. 2016;44(D1):D279–85.
Katoh K, Standley DM. MAFFT multiple sequence alignment software version 7: improvements in performance and usability. Mol Biol Evol. 2013;30(4):772–80.
Price MN, Dehal PS, Arkin AP. FastTree 2--approximately maximum-likelihood trees for large alignments. PLoS One. 2010;5(3):e9490.
Kück P, Longo GC. FASconCAT-G: extensive functions for multiple sequence alignment preparations concerning phylogenetic studies. Front Zool. 2014;11(1):81.
Stamatakis A. RAxML version 8: a tool for phylogenetic analysis and post-analysis of large phylogenies. Bioinformatics. 2014;30(9):1312–3.
Nguyen LT, Schmidt HA, von Haeseler A, Minh BQ. IQ-TREE: a fast and effective stochastic algorithm for estimating maximum-likelihood phylogenies. Mol Biol Evol. 2014;32(1):268–74.
Lanfear R, Frandsen PB, Wright AM, Senfeld T, Calcott B. PartitionFinder 2: new methods for selecting partitioned models of evolution for molecular and morphological phylogenetic analyses. Mol Biol Evol. 2016;34(3):772–3.
Holovachov O. Metabarcoding of marine nematodes – evaluation of similarity scores used in alignment-based taxonomy assignment approach. BDJ. 2016;4:e10647.
Holovachov O. Metabarcoding of marine nematodes – evaluation of reference datasets used in tree-based taxonomy assignment approach. BDJ. 2016;4:e10021.
Quast C, Pruesse E, Yilmaz P, Gerken J, Schweer T, Yarza P, Peplies J, Glöckner FO. The SILVA ribosomal RNA gene database project: improved data processing and web-based tools. Nucl Acids Res. 2013;41(D1):D590–6.
Download references
Acknowledgements
OH acknowledges support from the National Genomics Infrastructure funded by Science for Life Laboratory, the Knut and Alice Wallenberg Foundation and the Swedish Research Council, and SNIC/Uppsala Multidisciplinary Center for Advanced Computational Science for assistance with massively parallel sequencing and access to the UPPMAX computational infrastructure. OH also acknowledges the use of public servers usegalaxy.org (Center for Comparative Genomics and Bioinformatics at Penn State, the Department of Biology and at Johns Hopkins University and the Computational Biology Program at Oregon Health & Science University) and galaxy.ncgas-trinity.indiana.edu (National Center for Genome Analysis Support, Pervasive Technology Institute at Indiana University) for analysis of some of the data. Sampling in the Skagerrak was conducted by OH using vessels (“Skagerak” and “Oscar von Sydow”) and facilities of the Sven Lovén Centre for Marine Sciences in Kristineberg. Further thanks are to Dr. Sarah Atherton (Swedish Museum of Natural History) who collected samples containing Euchromadora sp. and Symplocostoma sp. KMK thanks Dr. Christoph Held, the Alfred Wegener Institute, and the scientists and crew of the R/V Polarstern PS 96 cruise, which provided samples used in this work. KMK also thanks Deb Crocker and Robert Griffin for assistance with the University of Alabama High-Performance Computing cluster. Dr. Andreas Hejnol (SARS Centre, University of Bergen), Dr. Philipp Schiffer (CLOE, University College London), and Dr. Christopher Kraus (Zoological Institute, Universität zu Köln) kindly provided unpublished transcriptome data. The authors gratefully acknowledge use of the resources of the Alabama Water Institute at The University of Alabama.
AS received funding from the Virginia Military Institute to support field work, identification of specimens, and manuscript preparation. Nematode sampling and transcriptome sequencing was supported primarily by two grants to OH from the Swedish Taxonomy Initiative, Artdatabanken: “Systematics of Swedish free-living nematodes of the orders Desmodorida and Araeolaimida” (Dha 2013–140) and “Systematics of poorly known marine nematodes of the class Chromadorea from Sweden” (SLU.dha.2017.4.3–102), and by a grant from Riksmusei Vänner: “Transcriptome sequencing of marine nematodes.” KMK received funding from The University of Alabama to support field work, lab work, transcriptome sequencing, data analysis, and manuscript preparation.
Author information
Authors and affiliations.
Department of Biology, Virginia Military Institute, 301B Maury-Brooke Hall, Lexington, VA, 24450, USA
Ashleigh B. Smythe
Department of Zoology, Swedish Museum of Natural History, Box 50007, SE-104 05, Stockholm, Sweden
Oleksandr Holovachov
Department of Biological Sciences and Alabama Museum of Natural History, The University of Alabama, Campus Box 870344, Tuscaloosa, AL, 35487, USA
Kevin M. Kocot
You can also search for this author in PubMed Google Scholar
Contributions
KMK, OH, and AS collected specimens, OH and AS identified specimens, KMK and OH performed RNA extraction and library preparation, and KMK analyzed the data. All authors contributed to the writing of the manuscript and approved the final version prior to submission.
Corresponding author
Correspondence to Kevin M. Kocot .
Ethics declarations
Ethics approval.
All specimens used in this study were collected from wild populations. Field work was conducted in accordance with local regulations. Antarctic nematodes and the kinorhynch were collected during R/V Polarstern cruise PS96 with permission from the Alfred Wegener Institute, Germany. No permits were required to collect any of the other taxa sequenced in this study.
Consent for publication
Not applicable.
Competing interests
The authors declare that they have no competing interests.
Additional information
Publisher’s note.
Springer Nature remains neutral with regard to jurisdictional claims in published maps and institutional affiliations.
Additional files
Additional file 1:.
Figure S1. Phylogenetic position of 18S rDNA fragment (contig Locus_123_Transcript_1/1) extracted from Heterorhabditis indica transcriptome dataset ( http://insilico.iari.res.in/hindica/ ). (PDF 46 kb)
Additional file 2:
Table S1. Names, classification (family and order), accession number (BioProject) or download link, and source/citation for publicly available genomes and transcriptomes of nematodes used in phylogenetic analysis. Classification is based on [ 1 ] with modifications [ 2 , 3 ]. Table S2. Names and origin data or reference for publicly available genomes, transcriptomes or proteomes of non-nematode (outgroup) taxa. (DOCX 22 kb)
Rights and permissions
Open Access This article is distributed under the terms of the Creative Commons Attribution 4.0 International License ( http://creativecommons.org/licenses/by/4.0/ ), which permits unrestricted use, distribution, and reproduction in any medium, provided you give appropriate credit to the original author(s) and the source, provide a link to the Creative Commons license, and indicate if changes were made. The Creative Commons Public Domain Dedication waiver ( http://creativecommons.org/publicdomain/zero/1.0/ ) applies to the data made available in this article, unless otherwise stated.
Reprints and permissions
About this article
Cite this article.
Smythe, A.B., Holovachov, O. & Kocot, K.M. Improved phylogenomic sampling of free-living nematodes enhances resolution of higher-level nematode phylogeny. BMC Evol Biol 19 , 121 (2019). https://doi.org/10.1186/s12862-019-1444-x
Download citation
Received : 10 December 2018
Accepted : 27 May 2019
Published : 13 June 2019
DOI : https://doi.org/10.1186/s12862-019-1444-x
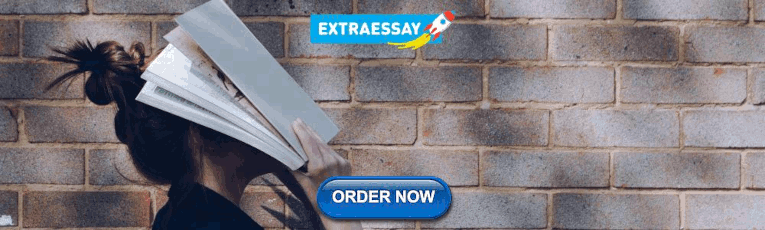
Share this article
Anyone you share the following link with will be able to read this content:
Sorry, a shareable link is not currently available for this article.
Provided by the Springer Nature SharedIt content-sharing initiative
- Phylogenomic
- Free-living
BMC Ecology and Evolution
ISSN: 2730-7182
- General enquiries: [email protected]
- Open access
- Published: 10 May 2021
Screening the nematicidal potential of indigenous medicinal plant extracts against Meloidogyne incognita under lab. and greenhouse conditions
- Hosny Kesba 1 ,
- Abdullah Abdel-Rahman 1 ,
- Samy Sayed ORCID: orcid.org/0000-0002-7002-568X 2 &
- Al-Sayed Al-Sayed 1
Egyptian Journal of Biological Pest Control volume 31 , Article number: 81 ( 2021 ) Cite this article
2254 Accesses
5 Citations
Metrics details
The root-knot nematode, Meloidogyne incognita , causes a high damage and yield decrease for many economic plants. The need for non-systemic effective new approaches and environmentally friendly methods for controlling the nematodes has directed research to some new and safe agrochemicals found in medicinal plants as new viable management options.
In laboratory experiments, solidago and periwinkle aqueous and ethanolic extracts achieved high J2 mortality (%) concerning different dilutions; however, aqueous extracts were more effective for mortality than ethanolic extracts. Also, there was a direct relationship between the nematicidal activity of these extracts with both concentration and time of application. Inhibition of egg hatching by Periwinkle extracts was higher than that of solidago. Moreover, the nematicidal activity of tested extracts against J2 decreased significantly with prolonged storage time at + 5 °C, while did not with stored frozen at – 5 °C for 12 months. Periwinkle and solidago extracts killed the non-target organisms, i.e., rotifers and free-living nematodes. Seventy-five and 90% of total phytochemicals recovered from periwinkle and solidago, respectively were nematostatic or nematicidal to nematode viability, egg hatch in vitro, and development and reproduction in vivo despite the method of application (foliar and soil drench). The antagonistic effects of solidago were more pronounced in soil drench than periwinkle concerning their concentrations and methods of application.
Solidago and periwinkle plant extracts showed important sources of effective control phytochemicals against M. incognita .
Botanicals can be used in in vitro and in vivo by different ways as one of the nonchemical approach strategies to manage and reduce plant-parasitic nematodes, especially in sustainable agriculture (Bridge 1996 ) by using their parts directly, their extracts, and compounds that possessing nematicidal activities, oilseed cakes, mature crop residues as organic amendments (Manju and Meena 2015 ). Some of the botanicals are already being exploited commercially in pest management and a rising trend towards organic farming (Zaidat et al. 2020 ).
In vitro, a lot of plant extracts showed high ovicidal and nematicidal effects on egg hatching and J2 survival of the root-knot nematode (RKN), M. incognita . Extracts from Nicotiana tabacum , Syzygium aromaticum , Piper betle , and Acorus calamus were found more effective in killing M. incognita , with an EC 50 which was 5–10 times lower than the EC 50 of the synthetic pesticides, chlorpyrifos, carbosulfan, and deltamethrin (Taniwiryono et al. 2009 ). The nematicidal effect of plant extracts could be higher than synthetic nematicides.
In vivo, (under greenhouse or field conditions) application of plant extracts reduced infection of RKN nematode and caused crop yield increase. These extracts were more effective than the nematicides used or in the same order or slightly less.
Dozens of phytochemical compounds that may be more active and eco-friendly, especially those came from medicinal and aromatic plants, e.g., serpentine, saponins, phenols, alkaloids, tannins, flavonoids, steroids, and cysteine proteinases have been reported for their antihelmintic effect against human, animal, and plant parasites (Rocha et al. 2017 ). The nematicidal effects of dried parts and boiled extract of Bidenspilosa were bioactive when re-evaluated on phytoparasitic nematodes after storing up to 12–18 months (Taba et al. 2012 ).
This study focused on the nematicidal potential of some medicinal plant extracts in the management of root-knot nematode in vitro and in vivo and their preservation on nematicidal activity, non-target organisms, and chemical composition.
Nematode culture
Pure stock culture of the RKN, M. incognita originally obtained from galled eggplant roots was established. Single egg-mass from previously identified females (Taylor et al. 1955 ) was used to inoculate healthy eggplants grown in (20 cm diameter) earthen pots filled with loamy sand soil. Three months after inoculation, plants were examined for nematode infection and reproduction. The culture was propagated and maintained on eggplant.
Plant extracts preparation
The effect of aqueous and ethanolic extracts of 13 medicinal plants was evaluated directly or after storage periods for lethal concentrations and toxicity index on survival and hatchability of M. incognita and non-target organisms’ management under laboratory conditions (in vitro). Therefore, foliar spray and soil drench applications of solidago and periwinkle, which achieved the highest mortality percentages than the other tested plant species were carried out in the greenhouse (in vivo) on infected sunflower plants.
Aqueous extracts
Twenty-five grams of air-dried leaves of 13 medicinal plants listed in Table 1 were homogenized by grinding for 1 min using a blender to coarse particles (formation of extremely soft particles like a powder that may hamper better extraction was avoided by the solvent as described by Pandey and Tripathi 2014 ). Then, 1 L of tap water was added. The mix was transferred to a 2-L beaker and was shaken vigorously. Decoction process was done to extract water-soluble and heat-stable constituents, in which the mix was boiled for 10 min, cooled, and filtered using filter paper. This mix was kept frozen as a stock solution (1×) until use. The stock solution was diluted by adding tap water to prepare the diluted extracts. Seven extracts dilutions (1:2×, 1:4×, 1:8×, 1:16×, 1:30×) were prepared by adding sufficient tap water to the stock solution till reaching the required concentrations. The 5 dilutions were equivalent, respectively, to the 5 concentrations (12500, 6250, 3120, 1560, 780 mg dry weight/liter (mg D.Wt./L)).
Ethanolic extracts
Five grams of the previously mentioned medicinal plants’ air-dried leaves were homogenized to coarse particles using a blender, then added to 200 ml of ethyl alcohol 96% in a 1-L beaker, shaken for 24 h using a shaker at room temperature, and filtered using a filter paper. A rotary evaporator was used to evaporate the solvent (ethanol) under vacuum to prepare the crude extracts, which then were dissolved in 5 ml ethanol and added to 200 ml tap water + 1 ml tween 80 as a surfactant. The resulting solution was shaken and kept frozen as a stock solution (1×). This stock solution is equivalent to a concentration of 25 mg D.Wt./L. Only 4 dilutions (1/2×, 1/4×, 1/8×, and 1/16×) were prepared by adding tap water to the stock solution till reaching the required dilutions, equivalent to 12500, 6250, 3120, 1560 mg D.Wt./L concentrations, respectively.
In vitro tests
Approximately 800 newly hatched J2 of M. incognita were tested for survival after exposure to the mentioned plant extracts after 48 h. For each treatment, 5 replicates were prepared in test tubes and kept under room temperature conditions. Juveniles in tap water only were served as a check. Mobile and immobile nematode J2s were counted under the microscope. Dead (immobile) J2s gave different strange body shapes such as S, Curly shapes. High protozoan and metazoan activities were noticed after juveniles’ death. Also, there was great degeneration ‘shrinkage’ starting after the stylet base and along the esophagus of the dead juveniles. Reversible effects were not expected. Mortality percent was calculated by the following formula:
Calculating lethal concentrations and toxicity index for M. incognita J2
Data of mortality percentages (%) in vitro were input to LDP line software to calculate probit analyses according to Finney ( 1971 ), which was used to illustrate the relation between stimulus and response in toxicological studies. The toxicity index of each plant extract was determined according to Sun ( 1950 ) using the following formula:
Egg hatching inhibition
According to mortality rates of J2 in the last-mentioned in vitro survival test , only 2 aqueous extracts that caused the highest mortality rates were chosen to test their effect on egg hatching rates, those were solidago and periwinkle extracts. Full egg masses of M. incognita were teased from infected eggplant roots under the stereomicroscope. Four concentrations of the 2 extracts were tested; 500, 1000, 2000, and 4000 mg D.Wt./L. Each replication received 5 full egg masses in a test tube, 5 replicates for each treatment, and incubated under room temperatures. The check was egg masses in tap water only. One week later, replicates were examined under the microscope to count hatched J2s. Inhibition rates were calculated according to the following formula:
Effects on non-target organisms
Concentrations of 1000 and 2000 mg D.Wt./L of the periwinkle and solidago extracts were tested against 2 kinds of metazoans; Rotifers and free-living nematodes in vitro. Four replicates for each treatment were set and mortality percentages were calculated after 48 h. Each replicate contained a mix of approximately 80 free-living nematodes and 100 rotifer individuals. The population of free-living nematodes was obtained from a soil sample rich in organic matter. However, rotifers were obtained from a soil sample kept at room temperature for a month to increase rotifers counts. The mixed population in water was kept as a check.
Storage periods of aqueous extracts
Samples of freshly prepared stock of each of solidago and periwinkle leave extracts were stored either under freezing at – 5 °C for 1 year, cooled for 2 weeks or 2 months at + 5 °C, then they were re-evaluated for their nematicidal effect changes. The experiment procedures and mortality percentages were done as previously mentioned on M. incognita J2 in vitro.
Greenhouse experiments
Based on data obtained from the mortality in vitro tests on M. incognita J2s , solidago and periwinkle aqueous extracts were tested under greenhouse conditions for M. incognita control. Seeds of sunflower (Giza-102) plants were sown in 220 pots, each filled with 2 kg sandy clay soil (1:1, v:v). Two weeks later, pots were divided into two main groups which were treated as follows:
Foliar spray application
The aqueous extracts were applied as a foliar spray once. Foliar spray drift to the soil was avoided by covering it, using a tissue paper. The tested plant aqueous extracts concentrations were 500, 1000, and 2000 mg dry leaves/L (mg D.Wt./L). Each treatment contained 5 replicates. After 2 weeks of germination, plants were inoculated with 2000 M. incognita J2s/plant . The whole experiment was set and horticultural-maintained for 45 days after nematode inoculation. Only one factor was different for each of the 3 sub-groups, which was the time application, as follows: the first subgroup; extracts were foliar sprayed 1 week before nematode inoculation, the second sub-group; extracts were foliar sprayed simultaneously with nematode inoculation, and the third sub-group; extracts were foliar sprayed 1 week after nematode inoculation. Five replicates in each sub-group received the same treatments, except that instead of applying plant extracts, the synthetic pesticide formulation, Vydate® 24% SL (oxamyl) was foliar sprayed as a standard chemical nematicide (3 ml/L). Another 5 replicates were inoculated with nematode only and kept as a check.
Soil drench application
The aqueous extracts were applied as a soil drench once (100 ml/plant). The tested plant aqueous extracts (solidago and periwinkle dry leaves extracts) concentrations were 500, 1000, and 2000 mg dry leaves/L (mg D.Wt./L). This group contained 110 pots, each treatment contained 5 replicates. Inoculum level was 2000 J2 of M. incognita after 2 weeks of germination . One factor was different for each of the 3 sub-groups, which was the time of extracts application as follows: the first subgroup; 100 ml of extracts solution were applied as a soil drench for each pot 1 week before nematode inoculation, the second sub-group; 100 ml of extracts were applied simultaneously with nematode inoculation, and the third sub-group: 100 ml of extracts were applied 1 week after nematode inoculation. Five replicates in each sub-group were received the same treatments except that, instead of applying plant extracts, the synthetic pesticide formulation, Vydate® 24 % SL, was soil drenched as a standard chemical nematicide (0.1 ml/L). Another 5 replicates were inoculated by nematode only and kept as a check.
Nematode assay
Upon harvest, each pot was soaked in a plastic bucket filled with water until the root system could be easily separated. Each root system was weighed and stored in 5% formaldehyde in plastic jars. The soil suspension was quite stirred and then poured through a series of 60 and 325 mesh screens (Hooper et al. 2005 ). The bottom sieve was then poured onto a modified Baermann set and collected after 48 h. Hawksley counting slide was used to calculate the number of J2s in 1 ml of the suspension and then referred to the whole volume. The numbers of galls and egg-masses were counted directly on the root system of each replicate and the mean of each treatment was calculated and later the eggs were extracted (Boneti and Ferraz 1981 ). For calculating eggs per egg mass, 10 full egg masses from each replicate were chosen, gelatin matrix was dissolved using sodium hypochlorite (NaOCl) according to (Hussey and Barker 1973 ), and eggs were counted in 1 ml volume under the microscope. The final population (eggs + soil population) plotted in the formula RF = Pf/Pi, where RF is the reproduction factor, Pf the final population, and Pi the initial population (Oostenbrink 1966 ).
GC/MS/MS analysis
Sample preparation.
Five grams of grinded dried leaves of each plant (periwinkle and solidago) were added to 100 ml deionized water in a 250-ml flask. The decoction process was done as previously mentioned. The extract was filtered, using filter paper, centrifuged at 9000 rpm for 5 min to exclude any impurities, then it was lyophilized (freeze-dried).
Chromatographic analysis
Produced powder from the last step was analyzed using gas chromatography/mass spectrometry and gas chromatography/tandem mass spectrometry (GC/MS/MS). The analysis was carried out using a GC (Agilent Technologies 7890A) interfaced with a mass selective detector (MSD, Agilent 7000) equipped with a polar Agilent HP-5ms (5%-phenyl methyl polysiloxane) capillary column (30 m × 0.25 mm i. d. and 0.25 μm film thickness). The carrier gas was helium with a linear velocity of 1 ml/min. The injector and detector temperatures were 200 and 250 °C, respectively. The volume injected 1 μl of the sample. The MS operating parameters were as follows: ionization potential 70 eV, interface temperature 250 °C, and acquisition mass range 50–800.
The identification of components was based on a comparison of their mass spectra and retention time with those of the authentic compounds and by computer matching with NIST and WILEY library as well as by comparison of the fragmentation pattern of the mass spectral data with those reported in the literature (Dong et al. 2014 ).
Statistical analysis
Data were statistically analyzed one-way ANOVA according to the SPSS software package version 23. The differences between means were tested using Duncan’s multiple tests at the 5% significance level.
Effect of aqueous extracts on J2 mortality
Dry leaf powder extracts of 13 medicinal plant species were tested in a laboratory assay on M. incognita J2s’ mortality with 5 dilutions of each. In general, after 48 h of exposure, all extracts used had nematicidal action against M. incognita J2 depending on the plant species and rate of the aqueous extract dilution (Table 2 ). The percent of mortality decreased by increasing extracts dilutions, except that of solidago and periwinkle dry leaf extracts. However, the first 2 dilutions 1:2× and 1:4× were highly toxic in all extracts achieving 100% mortality. Leaf extracts of solidago and periwinkle displayed the highest toxicity in (1:16× and 1:30× dilutions) than the rest of medicinal extracts, although, the percentage of mortality decreased down to almost 70% at (1:30× dilution). A considerable decrease in J2 mortality appeared by (1:16× dilution), less than 50% of J2s were killed by Common mint, horsemint, geranium, and dill extracts. The least percentages of mortality at (1:30× dilution) were recorded by extracts of marjoram, chamomile, and geranium, which were almost similar to the acceptable natural death percentage in the check (without treatment).
The relative toxicity index was measured by calculating and comparing each J2’s mortality relative to the maximum percentage of mortality. The accumulative percentages of mortality were calculated as well and were the highest in periwinkle followed by solidago and the least was found in Fros dill. The toxicity index, as well as the accumulative percentage of mortality, followed the same trend where the highest toxicity index was gained by solidago extract, followed by periwinkle and marjoram, respectively. While dill recorded the lowest relative toxicity value.
Concerning the lethal concentration needed to achieve LC 50 , it was found that solidago recorded the lowest lethal concentration that achieved such criteria and it was almost 1/2:1/3 of most extracts concentrations, followed by periwinkle. Geranium and dill needed four times concentrations as much as solidago to attain LC 50 . LC 90 was found to be achieved by a concentration of almost 1 g D.Wt./L in solidago extract. The lowest lethal concentration for LC 90 was raised to 5 times in horsemint, 10 and 11 times in rosemary and thyme extracts, respectively. Solidago and periwinkle extracts recorded almost the same lethal concentration required to achieve LC 90 .
Effect of ethanolic extracts on J2 mortality
The ethanolic extracts of the previously mentioned medicinal plant species were tested on M. incognita J2s mortality at 3 dilutions. After 48 h exposure, the ethanolic dry leaf extracts of all medicinal species were found lethal to M. incognita J2s, regardless of the rate of dilution, although the mortality percentages differed according to plant species extract and dilution (Table 3 ). There was direct proportional relation to a great extent between mortality and dilution. Considerable high mortality rates were noticed at 1:2× dilution in most cases than 1:4× or 1:8× dilutions. Coriander, geranium, solidago, marjoram, basil, and periwinkle extracts achieved higher mortality in 1:4× dilution than that of 1:2× or 1:8× dilutions. The general decrease in J2 mortality was recorded in 1:8× dilution, when compared with the others. The accumulative mortality percentages of geranium, marjoram, and solidago were the highest meanwhile; horsemint, chamomile, and dill were the lowest. However, the toxicity index of solidago extract was in the lead of all extracts.
From the results, it seems that the aqueous extracts of dry leaves of medicinal species were much more efficient in being nematoxic or nematicidal to M. incognita J2s than ethanolic extracts in all dilutions and that was reflected positively on the least lethal concentrations required for LC 50 and LC 90 .
Effect of storage aqueous extracts against J2
Results of the previous in vitro experiment verified that extracts of solidago and periwinkle recorded the ultimate percentages of J2 mortality amongst all the tested aqueous extracts. So, the effect of storage conditions and temperature on extracts stability and activity and in turn, their immobilization activity was studied (Table 4 ). The storage time affected the extract’s capability on mortality. The nematoxic effects of extracts were match able to those of expected fresh after 14 days storage in the fridge at + 5 °C, but when the storage period was prolonged up to 60 days, the stability of the phytochemicals was drastically affected, which in turn minimized mortality in solidago aqueous extract treatments. That minimization was more pronounced at 1000 mg D.Wt./L concentration. The activity of periwinkle extract lower concentration was less effective than the higher one. The activity of extracts differed after 12 months of freezing conditions at – 5 °C. Periwinkle activity was stable achieving 92% mortality at 1000 mg D.Wt./L and heightened up to 100% at the higher concentration. The opposite was found to be the case in solidago extract lower concentration (78% mortality). The higher concentration was highly effective as much as expected fresh or 14 days cooled storage. It seems that J2s mortality retrogrades proportionally with the time of extract storage. The efficacy of treatment was improved by doubling the concentration but not with the time of storage.
Effect of aqueous extracts on egg hatching
Solidago and periwinkle aqueous extracts which were found to be the most efficient on M. incognita J2s mortality were selected to study their effect on hatching of egg masses at 3 concentrations after 7 days of exposure. Significant inhibitory effects on the numbers of hatched eggs when compared with the check were observed (Fig. 1 ). Also, significant differences were found between and within treatments. Periwinkle extract was significantly efficient in reducing the number of hatched eggs at all concentrations. Non-significant differences were found between the lowest and middle concentrations of periwinkle. There was a significant positive correlation between the concentration of each aqueous extract and the rate of inhibition. The highest rate of inhibition was recorded at periwinkle (98.9%), followed by solidago (87.5%) with the highest concentration (1000 mg D.wt./L). The lowest inhibitory effects were found in the case of the lowest concentration of solidago extract (23.4%).
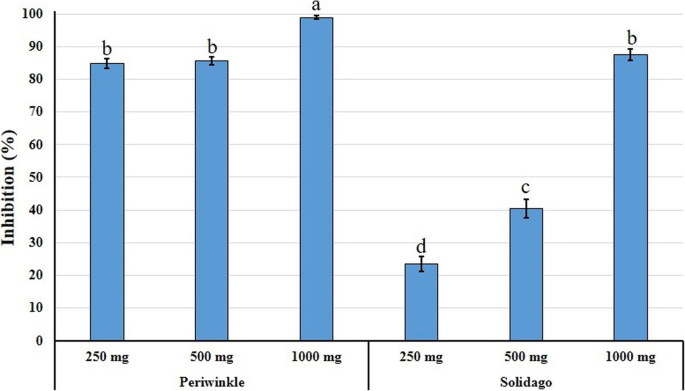
Efficacy of periwinkle and solidago aqueous extracts at three concentrations on egg hatching of M. incognita egg masses. Columns bear different letters that are significantly different at p < 0.05 according to Duncan’s multiple range tests
Effect of periwinkle and solidago aqueous extracts on non-target organisms
A further in vitro experiment was conducted to study the effect of solidago and periwinkle dry leaf aqueous extracts at 2 concentrations (1000, 2000 mg D.Wt./L) on the free-living nematode genera and rotifers, which were found co-inhabiting the soil with the root-knot nematodes. The recessive effects on both microorganisms were observed regardless of the type of plant extract as well as concentration; however, solidago extract was more efficient than periwinkle were obvious in Fig. 2 . Effects on rotifers were more retrograded than free-living nematodes. Mortality of rotifers after 48 h treatment was consistent in periwinkle leaf extract at the two used concentrations, which achieved more than 50% mortality. It is interesting to notice that percentage of mortality in solidago treatments was directly correlated with concentration, 66.5 at the lower and 84.7 at the higher. On the other hand, solidago aqueous extract interpreted almost similar mortality percentages at the lower and higher concentrations on free-living nematodes. Periwinkle extract at 1000 mg D.wt./L recorded mortality values on free-living nematodes 10% less than its higher concentration which was close to solidago extract concentrations.
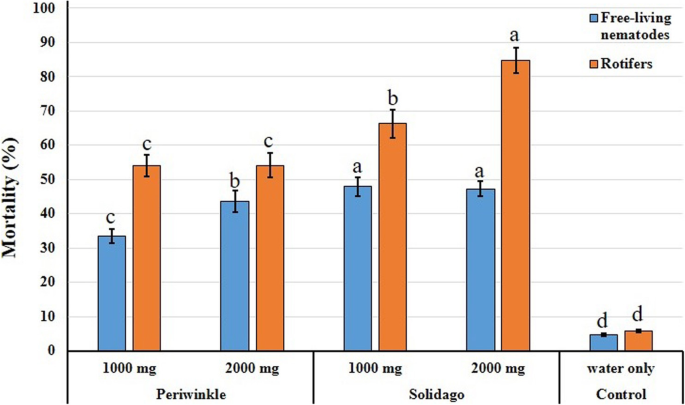
Efficacy of periwinkle and solidago aqueous extracts on the mortality of free-living nematodes and rotifers after 48 h in vitro . Columns of the same organism bear different letters are significantly different at p < 0.05 according to Duncan’s multiple range tests
Chemical content of solidago and periwinkle dry leaves
Quantitative and qualitative analysis of phytochemicals in dry leaves of periwinkle and solidago was carried out by GC-MS-MS. The leaf extract manifested four main phytochemical classes. Flavonoids, terpenoids, phenolics, and coumarins were highly present with different concentrations. Flavonoids were the most dominant in all the extracts, followed by the terpenoids, phenolics, and coumarins in the solidago leaf extract. Phenolic compounds were the maximum class found in periwinkle leaves (59.2%), followed by flavonoids (19.7%) and coumarins (5.2%). The minimum was piperazines (3.9%), glycosides (2.6%), and phytosterols (2.4%) in solidago leaves, respectively. Meanwhile, the lowest ratios were organic alcohol (4%), fatty acids (4.1%), alkaloids (3%), and sesquiterpenoids (3%) in periwinkle leaves. There were correspondingly prohibiting effects of the main chemical classes and the ratios on nematode activity, egg inhibition and development, and reproductively.
Effect of periwinkle and solidago aqueous extracts on RKN infecting sunflower
Potentials of periwinkle, solidago dry leaves aqueous extracts under 3 intervals (pre, with, and post-inoculation), 2 methods of application (foliar spray, soil drench) with 100 ml of each of the concentrations (500, 1000, 2000 mg D.Wt./L), and the comparable nematicide Vydate® 24% SL on M. incognita in greenhouse experiment was detected and illustrated in Tables 5 and 6 .
Apropos of pre-inoculation, periwinkle, and solidago extracts significantly suppressed the numbers of formed galls, egg masses, and eggs/egg mass. Except for periwinkle extracts, the numbers of eggs exceeded the nematode check and consequently reflected on nematode reproduction, which was more or less the nematode check. Solidago treatment concentrations performed significant impressive reductions in all nematode criteria and exhibited smashup in nematode final population and buildup. Obvious direct proportional effects were noticed among solidago concentration. Very few eggs were laid in the highest sprayed concentration (2000 mg D.Wt./L) achieving the lowest buildup (Table 5 ).
As for inoculation abreast with foliage spray, all treatments concentration imposed significant smashing reductions on all nematode criteria either intra- or interspecific treatments except the foliar sprays of solidago at 500, 1000 mg D.Wt./L at the number of deposited eggs and buildup, where the nematode was able to fold only once with non-significant differences with the check. Periwinkle concentrations were the most invincible where the least buildup rates were performed.
The post-inoculation treatment followed the same trend with the abreast one, all treatments were significantly effective in reducing numbers of galls, egg masses, final population, and the subsequent buildup and egg production when compared with the check. Solidago and periwinkle at 1000, 2000 mg D.Wt./L outmatched Vydate® 24% SL.
Concerning the foliar spray treatment, Vydate® at all intervals, the nematode galls, egg masses, and fecundity were sharply declined. Values of buildup were eliminated with significant differences where the nematode was not able to fold even once in pre- and post-inoculations.
Efficacies of the aqueous extracts of periwinkle and solidago on M. incognita at different intervals and same concentrations as previously mentioned and treated as a soil drench to sunflower plants are given in Table 6 . It contended in general that soil drench applications were more effective in reducing nematode criteria than foliar spray as measured by gall formation, egg masses fecundity, and buildup. Referring to the used concentrations, most if not all extracts concentrations were highly suppressive for nematode parameters. Solidago extracts surpassed to great extent periwinkle extracts at all intervals and concentrations. Controvert effects were noticeable in periwinkle extracts at 500, 2000 mg D.Wt./L at pre- and post-inoculation intervals, where the nematode was able to fold more than once as compared with the untreated inoculated check. The detersive effects of Vydate® were more malignant when treated as soil drench than foliar spray and in particular when treated, with inoculation, no J2s were able to invade the roots.
GC/MS/MS analysis indicated the presence of phytochemicals belonging to the following classes: phenols, flavonoids, triterpenoids, coumarins, alkaloids, and glycosides. groups with different concentrations. Solidago and periwinkle extracts were in the lead of all the 13 tested extracts in achieving the highest percentages of mortality. Ethanol extracts were effective in increasing mortality to M. incognita J2s but not as much as aqueous extracts. This may be due to the polarity of the solvent; the polarity of water is higher than that of ethanol. Consequently, higher antioxidants and phenols were extracted using water. Ng et al. ( 2020 ) reported that polar solvents could extract higher amounts of antioxidants and phenolic compounds, which increase the radical scavenging activity of the extracts. Neeraj et al. ( 2017 ) reported that alcoholic extracts showed a high activity in immobilization of M. incognita J2s and egg hatching inhibition in vitro. That may be because they used high concentrations.
The nature of medicinal plant structure and their derivatives have been discussed extensively but the mode of action of most nematicidal phytochemicals is still ambiguous. Periwinkle achieved more inhibitory effects on egg hatchability than solidago extract. That inhibition was proportionally correlated with concentration increase. It is well known that periwinkle extracts are rich in alkaloids. Extracts that contain alkaloids were found to have ovicidal property against Meloidogyne eggs (Adegbite 2003 ). Also, Alkaloids may act on the central nervous system and cause paralysis (Roy et al. 2010 ), which gave nematicidal effects. Important notice should be considered when comparing the efficacy of ethanolic and water extracts; solidago extracts with both solvents were the most toxic on J2. However, Periwinkle extract nematicidal effects were pronounced only in the aqueous extract and reduced greatly in the ethanolic one. Herein, the nematicidal and ovicidal effects of periwinkle extract are basically due to the water-soluble fractions.
Solidago aqueous extract had a higher percentage of compounds known with their nematicidal activity than periwinkle extract; such as terpenoids (Ohri and Pannu 2009 ), glycosides (Pronar 1983 ), and piperazines. Actually, for a long time, piperazine derivatives are used as antihelmintic drugs for humans (Shafei et al. 1955 ).
There is a real need for fractionation of the constituents of the extract to test each compound individually. However, one can generalize that the bioactivity against nematodes of each extract follows a multi-site mode of action. This is simply because that there is a large number of compounds in each extract, and these compounds have different functional groups with different modes of action.
Flavonoids, low molecular weight secondary metabolites have diverse functions including defense and auxin transport inhibition, and are implicated in resistance to both sedentary and migratory nematodes (Baldridge et al. 1998 ). Also, hypersensitive response and accumulation of the phytoalexin glyceollin, a product of isoflavonoids inhibit oxidation, respiration of M. incognita in vitro and accumulate adjacent to the head region of soybean cyst nematode in resistant root tissues (Kaplan and Keen 1980 ).
Phenolic compounds interfere with the energy generation mechanism by uncoupling the oxidative phosphorylation and interfere with glycoprotein of the cell surface of the parasite and cause death (John et al. 2009 ). Glycosides nematicidal effects are due to their function as a cholinesterase inhibitor that prohibits the normal buildup of nematode (Pronar 1983 ).
It has been found that prolonging the time of storage of extracts affects their stability and minimizes activity on nematode mortality, which was also influenced by the extract type. Aqueous extracts of periwinkle and solidago could be stored for 12 months without significant loss of nematicidal activity against M. incognita . The boiled extracts of Bidenspilosa could be stored for 12–18 months without loss of nematoxic activity (Taba et al. 2012 ).
Periwinkle and solidago extracts showed toxic effects on the free-living nematode and rotifers in vitro. Logically it may be lower than that of the synthetic nematicides but plant extracts are still considered xenobiotics and could alter the normal activity of the microfauna in the soil. Storage experiment revealed that bioactive compounds in these extracts were stable only at freezing temperatures, so biodegradation of these extracts in the soil is expected in a short period.
The greenhouse results emphasized the toxicity action of periwinkle and solidago extracts either foliar spray or soil drench treatment under different intervals on M. incognita parameters. The negative effects of solidago were more pronounced than periwinkle in most concentrations, method as well as the time of application. The nematicidal phytochemicals represented 90% of the total phytochemicals recovered from solidago meanwhile, that percentage was 75% for periwinkle. The nematode reduction outcomes may be due to the toxic contact action to the nematode juveniles and to the small molecular weight phytochemicals, which absorbed by roots easily that raise the plant resistance against nematodes or may have systemic nematicidal action as proven by pre-, with, and post-inoculation applications. That systemic nematicidal action may be attributable to the high ratios of phytochemical components (Archana and Parasad 2014 ) on other plants with different concentrations. Flavonoids could help in plant resistance against nematodes by affecting chemotaxis towards roots and interfere with functions in nematode reproduction (Chin et al. 2018 ). They added that the mechanism of these effects is still unknown. This can interpret the decline in reproduction factor and number of galls due to the application of both extracts. The medicinal plant extractions treated as soil drench showed significant reducing effects and nematicidal activity to M. incognita criteria (El-Nagdi and Youssef 2013 ). The described effects on M. incognita were achieved by using plant extracts with low concentrations under lab and greenhouse conditions which started with 0.5 g/l and did not exceed 2 g/l of the dry leaves. Comparing the used concentrations with the chemical nematicides may minimize the coast effectiveness. Solidago and periwinkle might be promising candidates of nematode management tactics.
Extracts of solidago and periwinkle leaves might be promising sources of phytochemicals that have nematicidal activity.
Availability of data and materials
All data generated or analyzed in this study are available in this published manuscript.
Abbreviations
Root-knot nematode
Dry weight/liter
Gas chromatography/mass spectrometry and gas chromatography/tandem mass spectrometry
Analysis of variance
Median lethal concentration
Ninety lethal concentration
Adegbite AA (2003) Comparative effects of Carbofuran and water extract of Chromolaena odorata on growth, yield and food components of root-knot nematode-infested soybean [ Glycine max (L.) Merrill]. Ph. D thesis, University of Ibadan, Ibadan
Google Scholar
Archana US, Parasad D (2014) Management of Plant-parasitic Nematodes by the Use of botanicals. Ann Plant Protec Sci 10(2):360–364 https://doi.org/10.4172/2329-955X.1000116
Baldridge GD, O’Neill NR, Samac DA (1998) Alfalfa ( Medicago sativa L) resistance to the root- lesion nematode, Pratylenchus penetrans : defense-response gene mRNA and isoflavonoidphytoalexin levels in roots. Plant Mol Biol 38(6):999–1010. https://doi.org/10.1023/A:1006182908528
Article CAS PubMed Google Scholar
Boneti JIS, Ferraz S (1981) Modificação do método de Hussey & Barker para extração de ovos de Meloidogyne exigua em cafeeiro. Fitopatol Bras Abst 6:553
Bridge J (1996) Nematode management in sustainable and subsistence agriculture. Annu Rev Phytopathol 34(1):201–225. https://doi.org/10.1146/annurev.phyto.34.1.201
Chin S, Behm CA, Mathesius U (2018) Functions of flavonoids in plant-nematode interactions. Plants 7(85):1–17 https://doi.org/10.3390/plants7040085
Dong L, Li X, Huang L, Gao Y, Zhong L, Yuanyuan Z, Zuo Y (2014) Lauric acid in crown daisy root exudate potently regulates root-knot nematode chemotaxis and disrupts Mi-flp-18 expression to block infection. J Exp Bot 65(1):131–141 https://doi.org/10.1093/jxb/ert356
El-Nagdi WE, Youssef MMA (2013) Comparative efficacy of garlic clove and castor seed aqueous extracts against the root-knot nematode, Meloidogyne incognita infecting tomato plants. J Plant Prot Res 53(3):285–288 https://doi.org/10.2478/jppr-2013-0042
Article Google Scholar
Finney DJ (1971) Probit analysis, 3rd edn. Cambridge Univ. Press, Cambridge, p 333
Hooper DJ, Hallmann J, Subbotin SA (2005) Methods for extraction, processing and detection of plant and soil nematodes. In: Luc M, Sikora RA, Bridge J (eds) Plant parasitic nematodes in subtropical and tropical agriculture. CABI Publishing, Wallingford, pp 53–86
Chapter Google Scholar
Hussey RS, Barker KR (1973) A comparison method of collecting inocula for Meloidogyne spp. including a new technique. Plant Dis Rep 57(12):1025–1028
John S, Chitra KR, Nambisan B, Mohandas C (2009) Nematicidal action of Cassava cyanogenson root-knot nematode ( Meloidogyne incognita ). Indian J Nematol 11:57–60
Kaplan DT, Keen NT (1980) Mechanisms conferring plant incompatibility to nematodes. Revue Nematol 3(1):123–134
Manju P, Meena S (2015) Antinemic properties of the botanicals. IJSN 6(2):125–134
CAS Google Scholar
Neeraj GS, Kumar A, Ram S, Kumar V (2017) Evaluation of nematicidal activity of ethanolic extracts of medicinal plants to Meloidogyne incognita (Kofoid and White) chitwood under lab conditions. Int J Pure App Biosci 5(1):827–831. https://doi.org/10.18782/2320-7051.2525
Ng ZX, Samsuri SN, Yong PH (2020) The antioxidant index and chemometric analysis of tannin, flavonoid, and total phenolic extracted from medicinal plant foods with the solvents of different polarities. J Food Process Preserv 44(9):e14680
Article CAS Google Scholar
Ohri P, Pannu SK (2009) Effect of terpenoids on nematodes: a review. J Environ Res Dev 4:171–177
Oostenbrink M (1966) Major characteristics of the relation between nematodes and plants. Meded Land- bouwhogeschool Wageningen 66:3–46
Pandey A, Tripathi S (2014) Concept of standardization, extraction and pre phytochemical screening strategies for herbal drug. J Pharmacogn Phytochem 2(5):115–119
Pronar OG (1983) Control of nematodes. In: The natural history of nematodes. Library of Congress cataloging in Publication Data by Prentice. Hall, Inc, Engle- wood, pp 289–298
Rocha TL, Soll CB, Boughton BA, Silva TS, Oldach K, Firmino AA, Silva LP, Polez VLP, Pelegrini PB, Bacicb A, Grossi-de-Sa M, Roessner U (2017) Prospection and identification of nematotoxic compounds from Canavalia ensiformis seeds effective in the control of the root knot nematode, Meloidogyne incognita . Biotechnol Res Innov 1(1):87–100 https://doi.org/10.1016/j.biori.2017.10.003
Roy H, Chakraborty A, Bhanja S, Nayak BS, Mishra SR, Ellaiah P (2010) Preliminary phytochemical investigation and anthelmintic activity of Acanthospermum hispidum DC. J Pharm Sci Technol 2(5):217–221
Shafei AZ, Nagaty HF, Rifaat MA, Salem S (1955) Piperazine as anthelmintic. Lancet 269:827–829
Sun YP (1950) Toxicity index an improved of comparing the relative toxicity of insecticides. J Econ Entomol 43(1):45–53 https://doi.org/10.1093/jee/43.1.45
Taba S, Ajitomi A, Shimabukuro Y, Yonaha M, Takara A, Nagamatsu Y, Moromizato Z (2012) Nematicidal activity of Bidens pilosa var. radiata boiled extracts on plant-parasitic nematodes and stability of the activity. J Weed Sci Technol 57(1):1–6 https://doi.org/10.3719/weed.57.1
Taniwiryono D, Berg H, Riksen JAG, Rietjens IMCM, Djiwantia SR, Kammenga JE, Murk AJ (2009) Nematicidal activity of plant extracts against the root-knot nematode, Meloidogyne incognita . Open Nat Prod J 2(1):77–85 https://doi.org/10.2174/1874848100902010077
Taylor AA, Dropkin VH, Martin GC (1955) Perineal pattern of root-knot nematodes. Phytopathology 45:26–30
Zaidat SAE, Mouhouche F, Babaali D, Abdessemed N, De Cara M (2020). https://doi.org/10.1186/s41938-020-00242-z ) Hammache M (2020) Nematicidal activity of aqueous and organic extracts of local plants against Meloidogyne incognita (Kofoid and White) Chitwood in Algeria under laboratory and greenhouse conditions. Egypt J Biol Pest Control 30(1):46
Download references
Acknowledgements
Not applicable.
This study was financed by Taif University Researchers Supporting Project number (TURSP-2020/92), Taif University, Taif, Saudi Arabia. This funder provided most chemicals used in plant extract and experimental bioassay.
Author information
Authors and affiliations.
Zoology and Agricultural Nematology Department, Faculty of Agriculture, Cairo University, Giza, 12613, Egypt
Hosny Kesba, Abdullah Abdel-Rahman & Al-Sayed Al-Sayed
Department of Science and Technology, University College-Ranyah, Taif University, B.O. Box 11099, Taif, 21944, Saudi Arabia
You can also search for this author in PubMed Google Scholar
Contributions
Methodology, H.K. and A.A-R.; formal analysis, S.S. and A.A.; investigation, H.K., A.A-R, and A.A.; resources, H.K. and S.S.; data curation, A.A.; supervision, A.A. and K.K; writing—original draft preparation, H.K, A.A-R., and A.A. writing—review and editing, H.K., S.S., and A.A. All authors have read and agreed to the published version of the manuscript.
Corresponding author
Correspondence to Samy Sayed .
Ethics declarations
Ethics approval and consent to participate, consent for publication.
This study does not contain any individual person’s data.
Competing interests
The authors declare that they have no competing interests.
Additional information
Publisher’s note.
Springer Nature remains neutral with regard to jurisdictional claims in published maps and institutional affiliations.
Rights and permissions
Open Access This article is licensed under a Creative Commons Attribution 4.0 International License, which permits use, sharing, adaptation, distribution and reproduction in any medium or format, as long as you give appropriate credit to the original author(s) and the source, provide a link to the Creative Commons licence, and indicate if changes were made. The images or other third party material in this article are included in the article's Creative Commons licence, unless indicated otherwise in a credit line to the material. If material is not included in the article's Creative Commons licence and your intended use is not permitted by statutory regulation or exceeds the permitted use, you will need to obtain permission directly from the copyright holder. To view a copy of this licence, visit http://creativecommons.org/licenses/by/4.0/ .
Reprints and permissions
About this article
Cite this article.
Kesba, H., Abdel-Rahman, A., Sayed, S. et al. Screening the nematicidal potential of indigenous medicinal plant extracts against Meloidogyne incognita under lab. and greenhouse conditions. Egypt J Biol Pest Control 31 , 81 (2021). https://doi.org/10.1186/s41938-021-00429-y
Download citation
Received : 24 February 2021
Accepted : 04 May 2021
Published : 10 May 2021
DOI : https://doi.org/10.1186/s41938-021-00429-y
Share this article
Anyone you share the following link with will be able to read this content:
Sorry, a shareable link is not currently available for this article.
Provided by the Springer Nature SharedIt content-sharing initiative
- Meloidogyne incognita
- Medicinal plant extracts
- Nematicidal activity
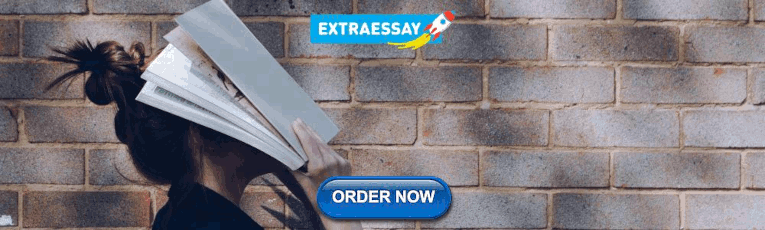
IMAGES
VIDEO
COMMENTS
In summary, with both field and laboratory work my PhD project has: 1) found associations between nematode trophic group abundance and vegetation cover types; 2) revealed the different response of grassland ecto-and endoparasitic nematodes to manipulated rainfall across a precipitation gradient; 3) quantified the herbivory index of a PPN
Entomopathogenic Nematodes for the Biological Control of Major Insect Pests on Tomato Darmstadt, Technische Universität Darmstadt, Jahr der Veröffentlichung der Dissertation auf TUprints: 2021 URN: urn:nbn:de:tuda-tuprints-190859 Tag der mündlichen Prüfung: 23.06.2021 Veröffentlicht unter CC BY-SA 4.0 International
A dissertation submitted to the Graduate Faculty of Auburn University in partial fulfillment of the requirements for the Degree of Doctor of Philosophy Auburn, Alabama May 2, 2020 ... Plant-parasitic nematodes are a major pathogen of turfgrass throughout the United States,
PLANT-PARASITIC NEMATODES FROM SOME EXOTIC PLANTS IN BELGIUM Huu Tien Nguyen Student number: 01608112 Promoter(s): Prof. Dr. Bert Wim, Prof. Dr. Wilfrida Decraemer A dissertation submitted to Ghent University in partial fulfilment of the requirements for the degree of International Master of Science in Agro- and Environmental Nematology
North Dakota State University
correct nematode identification and, more to the point, how we define a nematode species. As pointed Plants 2020 , 9 , 1260; doi:10.3390 / plants9101260 www.mdpi.com / journal / plants
PhD thesis Environmentally friendly control methods against the southern root-knot nematode (Meloidogyne incognita CHITWOOD) in forced vegetables by Zoltán Mándoki Budapest 2012 View metadata, citation and similar papers at core.ac.uk brought to you by CORE provided by Corvinus University of Budapest
PhD thesis: Sustainable insect control in vegetables through optimized applications of entomopathogenic nematodes September 2013 Thesis for: Doctor of applied biological sciences: agricultural ...
PLANT-PARASITIC NEMATODES ON SUGARBEET IN NORTH DAKOTA AND MINNESOTA A Thesis Submitted to the Graduate Faculty of the North Dakota State University ... assistance, guidance, support, and patience throughout my graduate program and thesis writing. I also would like to thank and express my gratitude to my graduate committee members, Dr.
nematodes consists of two non-differentiated germ cell primordia enclosed in two epithelial cap cells, and located approximately 65% of the body length from the anterior end (Subbotin, Mundo-Ocampo, and Baldwin 2010). J2s infect the roots of plants, and cause plant tissue to re- differentiate into a nematode-induced feeding site from which ...
cyanobacteria have been reported to inhibit hatching and to cause immobility and mortality of juvenile. plant parasitic nematodes in vitro. Application of cyanobacteria in soil may reduce nematode ...
statistically significant associations of specific haplotypes and nematode resistance in this population. The work in this thesis confirms the likely importance of MHC genes in regulating resistance against gastrointestinal nematodes, thus supporting the use of MHC as a genetic marker of nematode resistance in selective breeding.
View PDF; Download full issue; ... N., 2016. Predatory behaviour of Fictor composticola Khan et al. and its potential for the management of nematode pests of button mushroom, PhD thesis CCS HAU, Hisar, pp. 72. ... control of root knot nematode (Meloidogyne incognita) with predatory nematode, Fictor composticola on cucumber, PhD thesis CCS HAU ...
Nematodes are highly abundant and globally the most diverse group of soil fauna, and their analysis indicated that nematode community analysis was a useful tool to predict functional changes in soil ecosystems. Nematodes are highly abundant and globally the most diverse group of soil fauna. Due to the lack of taxonomists, only a handful of studies exist on communities of free-living nematodes ...
The diversity of nematodes changes randomly among different trophic groups along with the altitude. Bacteriovorous nematodes are found to be more abundant than other trophic groups (Fig. 2 A).Nematode diversity was found to be highest at 1000-1500 m.a.s.l. as was true for bacterivorous nematodes at 1000 m.a.s.l. and as far as diversity of other nematode groups like predators, plant parasites ...
Nematode isolation and storage. Late instar larvae of T. molitor, G. mellonella and B. tryoni were used to bait EPNs from the soil.Tenebrio molitor larvae were purchased from BioSupplies (Sydney, NSW, Australia) and kept at 25 °C and 70% RH on wheat bran with some carrot slices on top. Individuals of G. mellonella were collected from the Western Sydney University apiary and reared at 37 °C ...
Application of Trichoderma spp. in controlling various phytopathogenic nematodes leading to improved plant health and yield is the current brainstorming session among the researchers. Discover the ...
1. Introduction. Worldwide, root-knot nematodes (Meloidogyne species) are the most destructive plant-parasitic nematodes (PPNs).They infest more than 2 000 host plants, including ornamentals, weeds, medicinal plants, and edible crops (Khalil, 2013; Bernard et al., 2017).Recent reports have shown losses in several crops such as tomato, soybean, grapes, citrus, potato, beetroot, peanuts, spinach ...
Background Nematodes are among the most diverse and abundant metazoans on Earth, but research on them has been biased toward parasitic taxa and model organisms. Free-living nematodes, particularly from the clades Enoplia and Dorylaimia, have been underrepresented in genome-scale phylogenetic analyses to date, leading to poor resolution of deep relationships within the phylum. Results We ...
PDF | Root-knot nematode is a serious pest of various plants worldwide. It infests more than three thousand plant species. ... PhD Thesis: Khyber Pakhtunkhwa Agricultural (KPK) University ...
Background The root-knot nematode, Meloidogyne incognita, causes a high damage and yield decrease for many economic plants. The need for non-systemic effective new approaches and environmentally friendly methods for controlling the nematodes has directed research to some new and safe agrochemicals found in medicinal plants as new viable management options. Results In laboratory experiments ...
Root-knot nematode, M. incognita alone, soil borne wilt fungus, F. oxysporum alone and M. incognita + F. oxysporum concomitantly inoculated had suppressive effects on growth of cucumber cv. Sania (Table 1, Table 2, Table 3), pathogens infestation either inoculated singly or concomitantly caused significant (P > 0.05) reduction in shoot length, root length, fresh shoot, root weight, dry shoot ...
Two (out of 708 collected) female S. nigrogilvum harbored one third-stage larva (infective larva) each in their thoraces, resulting in an infection rate of 0.28%. The first larva was 1,037.22 μm long by 29.92 μm wide (Fig. 1 A), while the second one was broken with a remaining body length of 710.32 μm long by 25.52 μm wide (Table 2).The mitochondrial genes (cox1 and 12S rRNA genes) of the ...