December 16, 2022
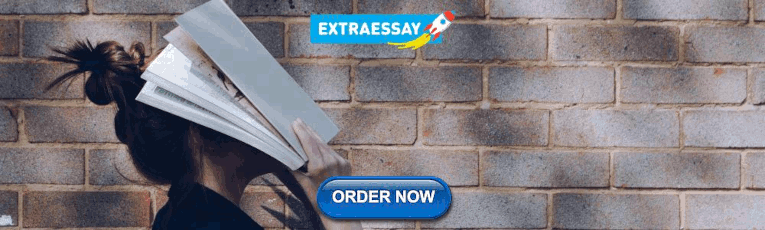
This Year’s Most Thought-Provoking Brain Discoveries
Neural circuits that label experiences as “good” or “bad” and the emotional meaninglessness of facial expressions are some standouts among 2022’s mind and brain breakthroughs
By Gary Stix
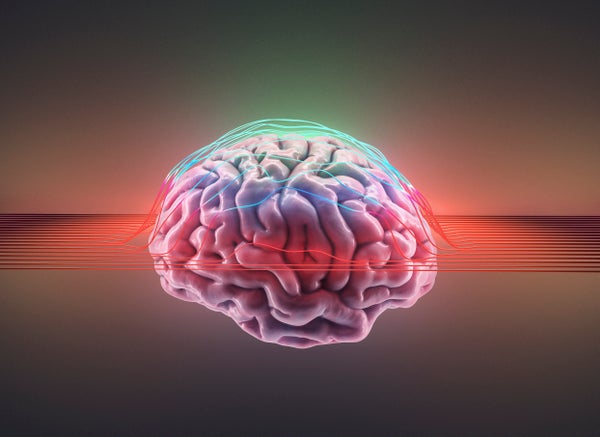
Jonathan Kitchen/Getty Images
Can the human brain ever really understand itself? The problem of gaining a deep knowledge of the subjective depths of the conscious mind is such a hard problem that it has in fact been named the hard problem.
The human brain is impressively powerful . Its 100 billion neurons are connected by 100 trillion wirelike fibers, all squeezed into three pounds of squishy flesh lodged below a helmet of skull. Yet we still don’t know whether this organ will ever be able to muster the requisite smarts to hack the physical processes that underlie the ineffable “quality of deep blue” or “the sensation of middle C,” as philosopher David Chalmers put it when giving examples of the “hard problem” of consciousness, a term he invented, in a 1995 paper.
This past year did not uncover a solution to the hard problem, and one may not be forthcoming for decades, if ever. But 2022 did witness plenty of surprises and solutions to understanding the brain that do not require a complete explanation of consciousness. Such incrementalism could be seen in mid-November, when a crowd of more than 24,000 attendees of the annual Society for Neuroscience meeting gathered in San Diego, Calif. The event was a tribute of sorts to reductionism—the breaking down of hard problems into simpler knowable entities. At the event, there were reports of an animal study of a brain circuit that encodes social trauma and a brain-computer interface that lets a severely paralyzed person mentally spell out letters to form words.
On supporting science journalism
If you're enjoying this article, consider supporting our award-winning journalism by subscribing . By purchasing a subscription you are helping to ensure the future of impactful stories about the discoveries and ideas shaping our world today.
Brain discoveries abounded in 2022—and will certainly continue in 2023. Here’s a look at a few prime pickings from what we published at Scientific American this year.
Your Brain Has a Thumbs-Up–Thumbs-Down Switch
When neuroscientist Kay Tye was pursuing her Ph.D., she was told a chapter on emotion was inappropriate for her thesis. Emotion just wasn’t accepted as an integral, intrinsic part of behavioral neuroscience, her field of study. That didn’t make any sense to Tye. She decided to go her own way to become a leading researcher on feelings. This year Tye co-authored a Nature paper that reported on a kind of molecular switch in rodents that flags an experience as either good or bad. If human brains operate the same way as the brains of the mice in her lab, a malfunctioning thumbs-up–thumbs-down switch might explain some cases of depression, anxiety and addiction.
Facial Expressions Do Not Convey What You’ve Been Taught about Someone’s Emotional Demeanor
Charles Darwin proposed that facial expressions are universal: a smile conveys happiness; a frown indicates sadness. He was wrong, suggests research published in recent years. Investigators found that innate expressions grounded in biology do not exist—and instead are highly variable. Neuroscientist Lisa Feldman Barrett warned in an essay that a recognition of Darwin’s fallacy has implications for AI facial recognition systems intended to detect emotions.
Your Kid May Be a Dandelion and an Orchid—And even a Tulip
Pigeonholing a child as either sensitive or resilient is probably a mistake. That child is not necessarily just an “orchid”—overly sensitive to adverse experiences—or a “dandelion”—relatively immune to such events. Newly arrived in the mix are “tulips,” children who experience modest effects from what’s happening around them. But even this floral triad might not suffice. Many kids are psychological mixes, mosaics, studies showed this past year . They display sensitivity to some but not all influences around them, depending on a particular situation.
If You See Something, It May Help You to Say Something
In a marriage of neuroscience and pedagogy, researchers tried to assess what a curriculum that emphasized the learning of spatial skills would do for kids. One example: an assignment that involved creating a map to track bears in the Blue Ridge Mountains. Kids at five Virginia high schools took courses, and their performance was matched against another group that received lessons without the spatial-learning component. The results of the research , published in August, showed that students in the spatial learning group improved not only spatial skills but also verbal abilities—figuring out a problem using words.

Fundamental Neuroscience Research
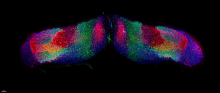
For well over a century, discoveries in basic neuroscience research have been the basis for our understanding of the nervous system and the foundation for developing treatments for neurological disorders. Insights into fundamental neuroscience (FN) have advanced at an ever-faster pace in the 21 st century, with remarkable novel discoveries in areas ranging from subcellular mechanisms of action to whole brain activities. FN generates key insights, drives innovation, and underlies many therapeutic breakthroughs that benefit humanity.
Background:
Neurology’s Stake in Foundational Neuroscience Research
Back to Basics: A call for fundamental neuroscience research
Discussion of Present and Future Plans For NINDS Support of Fundamental Neuroscience
The National Institute of Neurological Disorders and Stroke ( NINDS ) is planning a critical effort focused on advancing research in Fundamental Neuroscience (FN). To address this important and foundational aspect of neuroscience research, NINDS convened a Fundamental Neuroscience Working Group (FNWG) . FNWG held a series of meetings to discuss key issues and prepared a report to the NANDS Council with recommendations to inform NINDS approaches and plans to support and foster FN research. The report will be presented to NANDS Council on Wednesday September 6, 2023: NIH VideoCast - NANDS Council - September 2023 .
FNWG activities and materials can be found here: Fundamental Neuroscience Working Group (FNWG) . The FNWG's report on Advancing Fundamental Neuroscience Research (pdf, 915 KB) can be found here. Members of the public are encouraged to submit comments on the report, council presentation or any points related to promoting FN research to [email protected] .
Do you have a cool image related to FN that you would like share?

If so, please include your image with the following information and submit to: [email protected]
- Description of image
- Credit of image
NOTE: Image should be in a jpg, jpeg, gif, png or pdf format.
Resources and Tools Animal Models Resources available to neuroscience researchers interested in utilizing animals as models for nervous system function. Gene Expression The resources listed below are for gene expression-related information relevant to neuroscience research. Strategic Plan NINDS supports and performs a broad array of rigorous and important neuroscience research from fundamental studies of basic nervous system function to studies to improve treatments and prevent neurological disorders. NINDS Funding Strategy Current NINDS funding guidelines and payline. NIH BRAIN Initiative The Brain Research Through Advancing Innovative Neurotechnologies® ( BRAIN ) Initiative is aimed at revolutionizing our understanding of the human brain. Building Up The Nerve Neuroscience trainees are taken through the life cycle of a grant from idea to award at NINDS with the people who make it happen. Find an NINDS Program Director Please reach out to individual Program Directors or Program Managers for more information about specific opportunities or visit Find Your Program Director to learn more. Contact Us We would love to hear from you! Related FN Articles Inviting your input: fostering research in fundamental neuroscience Request for Information (RFI) on Advancing Research in Fundamental Neuroscience (Expired)
Fields of Study in Neuroscience
Reviewed by Psychology Today Staff
Neuroscience is a vast field of study containing a range of narrower subfields. Each involves a spotlight on the brain and other parts of the nervous system, connecting them to one or more zones of psychology and behavior—from thought processes to social interactions to mental illness.
Given how enmeshed the different aspects of mental life are, there is plenty of overlap between the different domains of neuroscience. Different branches can blend and feed into one another: Scientific research on cognition or emotions can be of value to neuroscientists who study psychiatric disorders, for instance.
Commonly recognized categories such as the ones below offer a sense of the breadth and diversity of neuroscience as an endeavor. Among the other fields of neuroscience are neuroanatomy, cellular and molecular neuroscience, and neurogenetics (the study of the nervous system’s genetic basis). Neuroscientists in each field are typically researchers with a doctoral-level degree (such as a Ph.D. or MD).
On This Page
- Cognitive Neuroscience
- Social Neuroscience
- Clinical Neuroscience
- Developmental Neuroscience
- Affective Neuroscience
- Behavioral Neuroscience
- Computational Neuroscience
Cognitive neuroscience investigates the neural mechanisms that underlie thinking and perception. It explores how information processing, which includes learning, remembering, deciding, and problem-solving, is made possible by the brain.
The scope of cognitive neuroscience includes how thought processes unfold at the cellular level—in specific neurons and the connections between them—as well as in the links between mental processes and larger brain regions and systems.
Cognitive neuroscientists explore how the brain gives rise to mental processes and abilities. To do so, they analyze measures of cognition and aspects of individual brains—from structural variation and differences in the function of certain brain areas down to the activity of specific neurons (as they encode, for example, the location of an object in space). Such research provides insights into which parts of the brain, for example, are especially active when someone is engaged in a cognitive function such as remembering or reading.
Examples of topics in cognitive neuroscience include the formation of memories at the level of neurons, how different brain areas collaborate to produce language ability , and how the brain’s perception of the world can be biased by factors such as motivation . Cognitive neuroscientists also focus on areas such as attention, learning, decision-making, and consciousness.
Social neuroscience examines the brain in the context of its connections to other people and the broader social world. It recognizes that humans are a highly social species and that the complexity of social interaction could help explain the evolution of the highly developed human brain.
Research in social neuroscience explores how a physical system gives rise to the kinds of relational processes that have long been observed in social psychology. It also seeks to describe the ways in which the brain and body themselves are affected by aspects of social life, such as social isolation or integration.
Social neuroscientists study the relationships between the nervous system and various aspects of social cognition and behavior. These include mental processes such as identifying someone as a member of a social group and trying to understand someone else’s perspective. Researchers use measurements of biological activity (such as functional activation of particular brain areas) as well as measures of thinking and behavior to investigate the associations between them and how they influence each other.
Social neuroscience has highlighted the existence of instantaneous brain activity related to social categorization and prejudice ; loneliness-related differences in how the brain represents the self and other people; and differences in the amygdalae of altruists and psychopaths . Other phenomena explored in social neuroscience include empathy, learning in social contexts, and group hierarchies.
Clinical neuroscience is an area of study that focuses on mental and nervous system disorders. These include disorders studied in psychiatry and clinical psychology, such as depression and anxiety disorders, as well as neurodegenerative conditions such as Alzheimer’s disease and multiple sclerosis. A major aim of neuroscientific research on clinical conditions is to contribute to improved methods of diagnosis, treatment, and prevention of disorder.
Researchers in clinical neuroscience analyze data on brain activation and other aspects of nervous system function and how they relate to various forms of mental illness or dysfunction. This may involve comparing patterns of brain activation in subjects who have clinical levels of dysfunction with those of healthy subjects. A clinical neuroscientist conducts scientific research but does not necessarily treat patients, whereas specialists in related clinical fields, such as neurology and neuropsychology, are involved in assessment and treatment.
Neuroscientific methods such as brain imaging have been applied to better understand various psychiatric, neurodevelopmental, and neurological conditions. Neuroscientists have observed, for instance, differences in how autistic children’s brains respond to incoming social information and in the activity of brain areas related to cognitive control in people with mood and anxiety disorders. Such findings could help inform theorizing about what causes symptoms in these conditions and how to target treatments.
Developmental neuroscience seeks to describe how the brain and nervous system form and change. The study of how age-related changes in the brain correspond with the development of individuals’ thinking and perception is called developmental cognitive neuroscience. Developmental neuroscientists explore both typical and atypical trajectories of nervous system development to better understand the bases of normal function and dysfunction.
Broadly speaking, developmental neuroscientists research how the anatomical form and functions of nervous systems develop within species, including both invertebrates and vertebrates. More specifically, developmental cognitive neuroscientists are interested in how cognitive processes that change as humans grow up—such as judgment and learning—correspond to aspects of the developing brain. They may, for example, present individuals of different ages, from young children to adults, with cognitive tasks and analyze how the results relate to differences in the activation of certain brain areas during the tasks.
Developmental neuroscientists have connected aspects of brain structure and function to various kinds of thinking and behavior over the lifespan. Neuroscientists have uncovered evidence that, for example, parts of the brain are more responsive to peer monitoring in adolescence than in adulthood; that less sleep in children is associated with lower volume in various brain areas ; and that the brain’s structure at age 6 is predictive of brain function and reasoning years later.
Affective neuroscience explores how the brain produces emotions. It identifies how particular structures, chemicals, and networks in the brain relate to affective states such as anger, fear, pleasure, and desire and how they give rise to complex emotional experiences. Affective neuroscientists use findings from humans as well as non-human animals with analogous emotional responses to better understand the nature of these responses.
Affective neuroscience involves observing how the activation of certain areas and networks of structures in the brain (as well as differences in brain structure) correspond to various emotional states, which researchers may evoke in the lab. Based on their findings, affective neuroscientists not only identify what is happening in the brain when individuals have particular sorts of emotional experiences, but also theorize and debate about what an emotion actually is —whether, for instance, there are deeply rooted “basic emotion” categories or whether emotions are more complex and consciously constructed.
Emotional experiences of all shades, and how the brain produces them, are the territory of affective neuroscientists. They have explored how specific brain chemicals and structures help produce emotional responses—such as the neurotransmitter dopamine and desire or the amygdala and automatic responses to threats (and less directly, feelings of fear). They have also illustrated that different parts of the brain work together to produce any particular emotional state.
Behavioral neuroscience, also called biological psychology, is the study of how the brain and the rest of the nervous system provide the foundation for behavior. It examines the neural basis of capacities such as thinking and perception, learning, emotion, and motivation. As such, behavioral neuroscience overlaps with a number of contemporary fields in neuroscience, such as cognitive neuroscience (focused on thinking and perception) and affective neuroscience (focused on emotions).
Behavioral neuroscientists investigate the links between the body and behavior in humans as well as non-human animals. They use a wide array of neuroscientific methods, from brain imaging in humans to the artificial stimulation of specific brain regions in rats. They also explore the role of factors that interact with the nervous system (such as hormones and drug consumption) in explaining behavior.
Studies of the neural mechanisms underlying craving and addiction, the formation of memories, fear conditioning and behavioral responses to threats, and impairments in cognitive abilities are all examples of research that falls under the category of behavioral neuroscience. Many experiments in behavioral neuroscience use models of nonhuman animal behavior as a way to better understand the neural basis of human behavior.
Computational neuroscience is an area of brain research that makes use of the power of computer modeling and mathematical tools to unpack how the brain works in all of its complexity. The approaches of computational neuroscience, which enable researchers to grapple with vast quantities of data about the nervous system, help them to develop explanations for how events unfold from the levels of chemicals and individual neurons up through the levels of neuronal networks and ultimately behavior and cognition.
Computational neuroscientists develop mathematical models and theories of neural function—accounts of the brain’s workings that can be tested based on data from studies of humans and nonhuman animals. Some use computer-based artificial neural networks, which use simplified representations of interconnected neurons, to help understand how information is handled by real networks in the brain. Computational neuroscientists also create models that directly incorporate real-world experimental data.
Neuroscientists have used computational methods to explore the processes underlying memory, attention, object recognition, and decision-making, among other cognitive capacities. Models of brain activity have been employed to study, for example, how the prefrontal cortex learns about new situations and how damage to it might impair this ability.
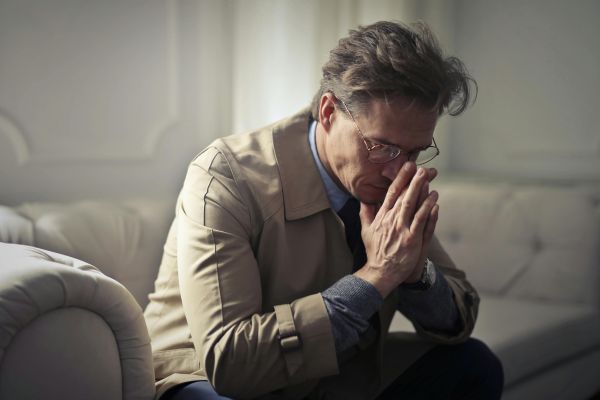
An abundance of evidence supports the relationship between anesthesia and memory loss. The severity and frequency of exposure to anesthesia could lead to mild cognitive impairment.
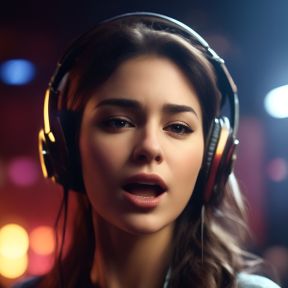
Music-induced pleasure relies on the engagement of both higher-order brain regions as well as some primitive reward-related areas.
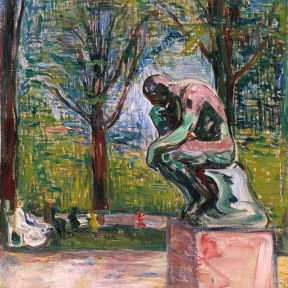
That little voice inside your head? The puzzle of consciousness would still be there without it. Here's why.
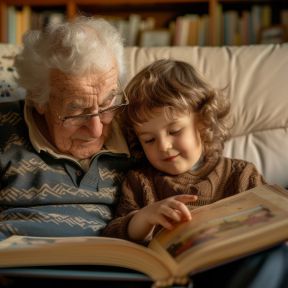
Is it better for kids to read books or spend time on screens? Research shows early reading for pleasure is a game-changer for healthy brain development. Screen time? Not so much.
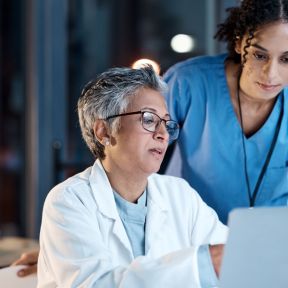
The time is now for the AI community to build explicit reasoning systems in service of implicit reasoning LLMs to maintain momentum in healthcare advances.
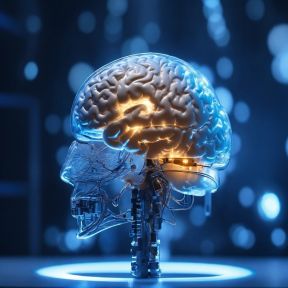
AI deep learning powers a brain-computer interface that enables humans to continuously control a cursor using thoughts.
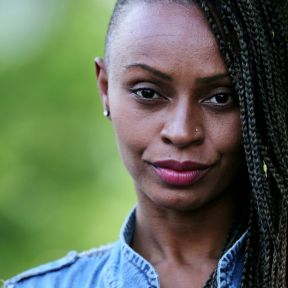
Left-brained? Right-brained? No, just...brained.
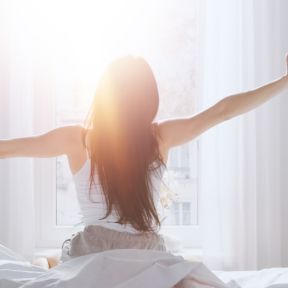
Sleeping in leads to cascades of negative effects that can interfere with cardiac and metabolic health and body weight regulation.
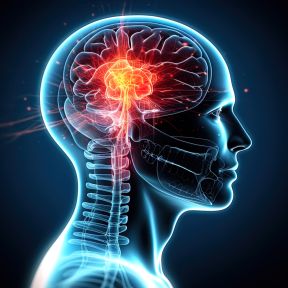
Chronic pain often involves nociplastic pain. I call it the “spreading pain” because it causes the discomfort to extend beyond the area of injury.
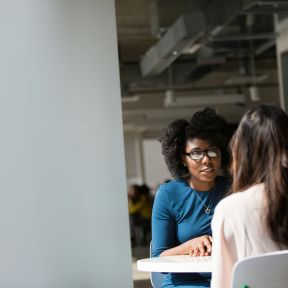
Most performance reviews are more about the reviewer than the direct report. Here are 6 strategies to enhance your reviews to be more effective.
- Find a Therapist
- Find a Treatment Center
- Find a Psychiatrist
- Find a Support Group
- Find Online Therapy
- United States
- Brooklyn, NY
- Chicago, IL
- Houston, TX
- Los Angeles, CA
- New York, NY
- Portland, OR
- San Diego, CA
- San Francisco, CA
- Seattle, WA
- Washington, DC
- Asperger's
- Bipolar Disorder
- Chronic Pain
- Eating Disorders
- Passive Aggression
- Personality
- Goal Setting
- Positive Psychology
- Stopping Smoking
- Low Sexual Desire
- Relationships
- Child Development
- Therapy Center NEW
- Diagnosis Dictionary
- Types of Therapy
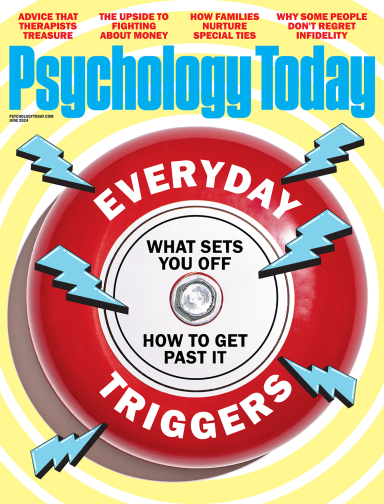
At any moment, someone’s aggravating behavior or our own bad luck can set us off on an emotional spiral that threatens to derail our entire day. Here’s how we can face our triggers with less reactivity so that we can get on with our lives.
- Emotional Intelligence
- Gaslighting
- Affective Forecasting
- Neuroscience

An official website of the United States government
The .gov means it’s official. Federal government websites often end in .gov or .mil. Before sharing sensitive information, make sure you’re on a federal government site.
The site is secure. The https:// ensures that you are connecting to the official website and that any information you provide is encrypted and transmitted securely.
- Publications
- Account settings
Preview improvements coming to the PMC website in October 2024. Learn More or Try it out now .
- Advanced Search
- Journal List
- HHS Author Manuscripts

Using Cognitive Neuroscience to Improve Mental Health Treatment: A Comprehensive Review
Jessica a. wojtalik.
Doctoral candidate at the University of Pittsburgh School of Social Work
Shaun M. Eack
Professor at the University of Pittsburgh School of Social Work and Department of Psychiatry
Matthew J. Smith
Associate professor at the University of Michigan School of Social Work
Matcheri S. Keshavan
Professor in the Harvard Medical School Department of Psychiatry
Mental health interventions do not yet offer complete, client-defined functional recovery, and novel directions in treatment research are needed to improve the efficacy of available interventions. One promising direction is the integration of social work and cognitive neuroscience methods, which provides new opportunities for clinical intervention research that will guide development of more effective mental health treatments that holistically attend to the biological, social, and environmental contributors to disability and recovery. This article reviews emerging trends in cognitive neuroscience and provides examples of how these advances can be used by social workers and allied professions to improve mental health treatment. We discuss neuroplasticity, which is the dynamic and malleable nature of the brain. We also review the use of risk and resiliency biomarkers and novel treatment targets based on neuroimaging findings to prevent disability, personalize treatment, and make interventions more targeted and effective. The potential of treatment research to contribute to neuroscience discoveries regarding brain change is considered from the experimental-medicine approach adopted by the National Institute of Mental Health. Finally, we provide resources and recommendations to facilitate the integration of cognitive neuroscience into mental health research in social work.
Mental health is an essential component of overall health and well-being ( World Health Organization, 2013 ), and mental illnesses—such as schizophrenia, depression, bipolar disorder, post-traumatic stress disorder (PTSD), and autism—are leading causes of disability in the U.S. ( U.S. Burden of Disease Collaborators, 2013 ). These mental health conditions are too often functionally disabling, impacting individuals’ ability to think clearly, live independently in the community, maintain meaningful interpersonal relationships, and achieve personal goals. Social workers are the largest group of mental health care providers in the U.S. ( Heisler & Bagalman, 2015 ) and have contributed to the development and testing of psychosocial interventions for people living with some of the most disabling mental health conditions (e.g., Anderson, Reiss, & Hogarty, 1986 ; Garland, 2013 ; Hogarty et al., 2004 ; Rapp, 1998 ; Stein & Test, 1980 ). Despite decades of focused research on pharmacological and psychosocial interventions to improve mental health—and despite numerous significant advances—the effectiveness of these interventions remains only moderately successful ( Bishop-Fitzpatrick, Minshew, & Eack, 2014 ; Mueser, Deavers, Penn, & Cassisi, 2013 ; Newby, McKinnon, Kuyken, Gilbody, & Dalgleish, 2015 ). Very few people with disabling mental illness achieve personally acceptable clinical and functional recovery, and even fewer return to levels of psychosocial functioning experienced prior to the onset of their condition ( Harvey & Bellack, 2009 ; Judd et al., 2008 ; McIntyre & O’Donovan, 2004 ). Recent advances in cognitive neuroscience research offer unique opportunities to improve the effectiveness of both pharmacological and psychosocial interventions for those confronted with mental health issues.
The integration of cognitive neuroscience and mental health research to increase the effectiveness of interventions that promote recovery from mental illness is a research priority ( National Institutes of Health, 2015 ), and social workers have a central role in such translational research efforts ( Brekke, Ell, & Palinkas, 2007 ). National Institute of Mental Health (NIMH) research priorities include investigating the neurobiological mechanisms and trajectories of mental health conditions to identify optimal therapeutic windows for intervention and possibly prevent illness onset. (For more information about the NIMH’s research priorities and associated funding announcements, see https://www.nimh.nih.gov/about/strategic-planning-reports/strategic-research-priorities/index.shtml .) In the current article, we review the emerging trends from cognitive neuroscience and brain plasticity research and provide examples of how these advances can be used by social workers and allied professions to improve mental health treatment (see Table 1 ). We encourage social work researchers, practitioners, and mental health educators to facilitate the integration of cognitive neuroscience by using this article to become familiar with the cognitive neuroscience vocabulary, introducing it into social work courses, and beginning to incorporate neuroimaging measures into their own research.
Key Points on Using Cognitive Neuroscience to Improve Mental Health Treatment
Note . NIMH 5 National Institute of Mental Health.
In 1990, U.S. President George H. W. Bush signed a proclamation designating 1990– 2000 as the “Decade of the Brain” ( Bush, 1990 ) to promote considerable neuroscience research efforts and raise public awareness of neurobiologically based conditions, such as schizophrenia and Alzheimer’s disease ( Goldstein, 1990 ). After nearly three decades, incredible progress has been made in the fundamental understanding of the human brain ( Insel & Landis, 2013 ; Jones & Mendell, 1999 ) and the neurobiological etiology of mental illness ( Charney, Buxbaum, Sklar, & Nestler, 2013 ; Goodkind et al., 2015 ). Indeed, many mental health conditions are now understood to involve numerous aspects of the brain and to follow a neurodevelopmental trajectory ( Ansorge, Hen, & Gingrich, 2007 ; Faludi & Mirnics, 2011 ; Keshavan, Anderson, & Pettergrew, 1994 ). Some of the most disabling mental health symptoms emerge in late adolescence and early adulthood ( Paus, Keshavan, & Giedd, 2008 ), which is a critical period of brain development ( Purves, White, & Riddle, 1996 ); the emergence of these symptoms has been shown to correspond with abnormal synaptic pruning and proliferation ( Faludi & Mirnics, 2011 ; Keshavan et al., 1994 ). This neurodevelopmental insult results in abnormal brain function ( Pantelis et al., 2003 ) and significant loss of gray matter volume ( Cannon et al., 2015 ), which underlie many of the signs and symptoms of mental illness (e.g., substantial cognitive challenges observed in individuals with schizophrenia; Fusar-Poli, Radua, McGuire, & Borgwardt, 2011 ; Rapoport, Giedd, & Gogtay, 2012 ).
Along with the evident neural basis and impact of many mental health conditions, the brain is also known to be “plastic.” Studies of neuroplasticity—the ability of the brain to adapt and change—suggest that the adult human brain has a remarkable capacity for strengthening and generating new neuronal connections to enhance daily functioning ( Bruel-Jungerman, Davis, & Laroche, 2007 ; Buonomano & Merzenich, 1998 ). Such findings have generated renewed therapeutic optimism for the possibility of greater recovery from mental health conditions that were previously thought to be characterized by static encephalopathy ( Goldberg, Hyde, Kleinman, & Weinberger, 1993 ). Thus, social workers have begun to integrate cognitive neuroscience methods into their research to understand and enhance the efficacy of their interventions (e.g., Eack et al., 2010 ; Garland, Froeliger, & Howard, 2015 ; Matto et al., 2013 ). For example, Eack, Newhill, and Keshavan (2016) demonstrated that individuals with schizophrenia who received a psychosocial treatment to improve their thinking skills had increased communication between frontal and temporal brain areas, which was associated with enhanced emotion processing. Such results suggest that frontotemporal neural communication may be an important treatment target for improving recovery. Moreover, one could conclude that cognitive training might be more effective if these interventions engaged clients in activities that would support enhanced communication between frontotemporal brain regions. Numerous social work investigators have written about the value of integrating cognitive neuroscience and clinical intervention research ( Farmer, 2008 ; Matto & Strolin-Goltzman, 2010 ; Matto, Strolin-Goltzman, & Ballan, 2014 ; Shapiro & Applegate, 2000 ).
Cognitive Neuroscience Basics
To understand the implications that cognitive neuroscience holds for informing mental health treatment, it is first essential to understand some basic knowledge about neuroscience and the brain. The field of cognitive neuroscience is directed toward the study of the neurobiological mechanisms that underlie mental processes and behaviors ( Frackowiak, 2004 ), and for the purposes of this article, we broadly include cognitive, affective, and clinical neuroscience domains under the cognitive neuroscience rubric. The brain is an incredible organ that can generally be defined as an integrated and complex information processing system that generates thoughts, emotions, and behaviors. The adult human brain is estimated to contain 100 billion neurons, which is comparable to the number of stars in the Milky Way galaxy ( Fischbach, 1992 ). Remarkably, all this processing power is packed into a mere 3 pounds of tissue (about 1300 cubic centimeters), and much of the brain’s function is being actively investigated and discovered.
Brain anatomy
The anatomy of the brain is broadly made up of gray matter, white matter, and cerebrospinal fluid. Gray matter comprises the cerebral cortex (i.e., the top and outer portions of the brain) and contains neuronal and glial cell bodies, dendrites, and unmyelinated axons (i.e., axons that do not have myelin containing sheaths). Unmyelinated axons are largely responsible for conscious and effortful mental processes ( Rosenzweig, Breedlove, & Watson, 2005 ), including memory and problem-solving. The cerebral cortex has a folded appearance, with the peaks known as gyri and the valleys referred to as sulci . White matter is located deeper in the brain and contains bundles of neuronal tracts that connect different areas of gray matter. These tracts encompass axons that are wrapped in myelin , which is an insulating fatty material that increases neuronal communication. White matter tracts interconnect and organize neuronal transactions in gray matter ( Rosenzweig et al., 2005 ). Among other functions, cerebrospinal fluid cushions the brain and fills the ventricles (i.e., fluid filled cavities) throughout the brain to protect it from injury during head movement.
The cerebral cortex is a primary focus of cognitive neuroscientists and is organized into five broad regions, or lobes—frontal, parietal, temporal, occipital, and limbic (see Figure 1 ). The frontal lobe is vital to a person’s ability to function within society, facilitating executive function (i.e., working memory), higher order social cognitive abilities (i.e., emotion regulation, theory of mind), self-awareness, moral reasoning, language, and voluntary movement ( Adolphs, 2001 ; Chayer & Freedman, 2001 ). The parietal lobe has a role in tactile and sensory processing. For example, spatial awareness, visuomotor actions (i.e., grasping an object), mathematical operations, imitation, and understanding intentions of others are cognitive abilities associated with the parietal lobe ( Dehaene, Piazza, Pinel, & Cohen, 2003 ; Fogassi & Luppino, 2005 ; Rizzolatti, Fogassi, & Gallese, 2001 ). The temporal lobe contains the primary auditory cortex involved in speech processing and the hippocampus involved in memory ( Squire & Zola-Morgan, 1991 ). Lower-order social cognition ( Adolphs, 2001 ; LaBar, Crupain, Voyvodic, & McCarthy, 2003 )—such as perceiving socially relevant information and recognizing emotions in faces—is also associated with the temporal lobe. The occipital lobe is the location of the primary visual cortex and is involved in visual processing. Finally, the limbic system and insular cortices ( Figure 1 ) are evolutionally older regions located deep in the brain and are central to basic human functioning. Physical and social pain are processed in the insula ( Craig & Craig, 2009 ; Kross, Berman, Mischel, Smith, & Wager, 2011 ), and impulsivity, reward, and emotions are associated with the limbic system ( McClure, Laibson, Loewenstein, & Cohen, 2004 ; Morgane, Galler, & Mokler, 2005 ). A basic knowledge of the brain’s functional anatomy is important for understanding how various neuroimaging methods assess brain structure and function. It is also important to remember that the brain is a vast and highly connected network, and that cognitive and behavioral functions are largely the result of the coordination of numerous brain regions that can span lobes.

Visualization of the broad regional organization of the human cerebral cortex.
Neuroimaging approaches
Neuroimaging methodologies are noninvasive techniques used in cognitive neuroscience to assess the structure and function of the human brain. Magnetic resonance imaging (MRI) is a technique for assessing brain structure that produces a high-spatial-resolution image of brain anatomy, including gray matter, white matter, and cerebrospinal fluid. MRI is one of the most commonly used neuroimaging techniques for assessing neuroanatomy. Common measures of brain function (or activity) include positron emission tomography (PET) and functional magnetic resonance imaging (fMRI). Both measure brain activation over a period of time by indirectly assessing blood flow while a participant is performing a cognitive task in the scanner. More specifically, fMRI measures the blood oxygenation level dependent (BOLD) signal and reflects changes in blood flow ( hemodynamic response ) that correspond to neural activity ( Huettel, Song, & McCarthy, 2008 ). PET scanning requires the injection of a radioactive tracer, which is then measured (as opposed to intrinsic blow flow) to obtain an assessment of brain function ( Huettel et al., 2008 ); PET is used less frequently due to the need to inject the radioactive tracer. Other neuroimaging techniques index neural communication patterns and can reflect either functional or structural connectivity throughout the brain ( Friston, 1994 , 2011 ; Hermundstad et al., 2013 ). For example, resting-state fMRI assesses functionally linked brain areas while the person is resting (i.e., not performing a task in the scanner; Van Den Heuvel & Pol, 2010 ). Similarly, task-based functional imaging studies measure correlated patterns of brain activity when a person is performing a task in the scanner ( Barch et al., 2013 ). In contrast, diffusion tensor imaging (DTI) is used to examine structural connectivity, which is a measure of how well white matter tracts connect with gray matter ( Sporns, Tononi, & Kötter, 2005 ). Together, these different neuroimaging methodologies provide powerful strategies for understanding how the brain is organized, how it functions when processing information, and how various regions communicate to support cognition and behavior. Perhaps most importantly, all of these measured parameters can be modified by mental health interventions ( Barsaglini, Sartori, Benetti, Pettersson-Yeo, & Mechelli, 2014 ; Messina, Sambin, Palmieri, & Viviani, 2013 ; Ramsay & MacDonald, 2015 ).
The Dynamic, Neuroplastic Brain
As neuroimaging methods rapidly advance, data increasingly indicate that the brain is malleable, neuroplastic, and reciprocally influenced by the social environment, leading to entirely new opportunities for social work interventions to enhance psychosocial functioning and recovery. For many years, neuroscientists steadfastly believed that once the brain reached its mature state in adulthood, the number of neural connections were fixed. However, early cognitive neuroscience research in rodents demonstrated that environmental enrichment increased neuronal connections, providing the first evidence of neuroplasticity ( Diamond, Krech, & Rosenzweig, 1964 ). Neuroplasticity is the capability of the brain to be flexible, dynamic, and adjust to the environment by creating, rebuilding, or strengthening neuronal connections, especially in response to learning or injury ( Bruel-Jungerman et al., 2007 ; Buonomano & Merzenich, 1998 ). In the 1980s, Taub (1980) advanced the understanding of neuroplasticity ( Schwartz & Begley, 2003 ) by revealing that neural connections could be regenerated in the somatosensory cortex of monkeys after the connections controlling one arm were severed. Taub and colleagues (1993) later applied these findings to rehabilitating neural connections important for daily living skills after a stroke using constraint-induced movement therapy. This therapy forces the reorganization of neural connections—often motor neurons—in the brain area damaged by stroke. Individuals treated with constraint-induced movement therapy practice movements using the impacted limb by constraining the unimpacted, contralateral appendage ( Taub, Uswatte, & Mark, 2014 ). Taub’s original findings provided impetus to study adult brain plasticity, and the last 40 years of research in this area has demonstrated convincing evidence that the adult brain is, in fact, capable of remarkable changes through the creation and adaptation of neural connections based on environmental experience ( Fuchs & Flügge, 2014 ).
People exist in an ever-changing physical and social environment that influences brain development and function, which has implications for mental health treatment research. Given this plasticity feature, the brain is always changing. For instance, individuals who develop a mental illness often significantly withdraw to an impoverished and isolated social life ( Hooley, 2010 ). Thus, exposure to social deprivation, neglect, and stress negatively influences the brain by modifying circuits (i.e., preventing the creation of new neuronal connections) responsible for cognitive function ( Lu et al., 2003 ). For example, experiencing chronic stress can be neurotoxic and inhibit factors important for neuronal health ( Pittenger & Duman, 2008 ). This effect of stress contributes to abnormal brain functioning and gray matter loss, and it has been observed to lead to cognitive impairment in people with depression ( Fossati, Radtchenko, & Boyer, 2004 ), schizophrenia ( Cannon et al., 2015 ), and autism ( Berger, Rohn, & Oxford, 2013 ). Within this special section on social work and neuroscience, the article “The Neuroscience of Resilience” by Hunter, Gray, and McEwan (in press) discusses the effects of early adversity and stress on the brain. As Hunter et al. note, it is increasingly clear that negative social environments and experiences induce adverse neuroplasticity, changing the brain for the worse (i.e., gray matter loss). These findings are particularly relevant for social work because they provide evidence of the powerful role that the social environment plays in brain development and function—an important assumption of the biopsychosocial model and prevention efforts.
Fortunately, supportive and enriched social environments can facilitate adaptive brain plasticity ( Davidson & McEwen, 2012 ; Keshavan, Mehta, Padmanabhan, & Shah, 2015 ) by strengthening or generating novel neural connections reflected in increased gray matter and/or brain activation. Research in healthy volunteers has provided some of the first evidence of adaptive neuroplasticity in adulthood through learning or training ( Driemeyer, Boyke, Gaser, Büchel, & May, 2008 ; Maguire et al., 2000 ). For example, participants who learned to juggle over a 3-month period displayed increased gray matter in the middle temporal gyrus and intra-parietal sulcus—which are involved in visuomotor and visuospatial processing— compared to a nonjuggling group ( Draganski et al., 2004 ). Regarding mental health, the principles of neuroplasticity are the driving force for cognitive remediation interventions ( Keshavan, Vinogradov, Rumsey, Sherrill, & Wagner, 2014 ; Morimoto, Wexler, & Alexopoulos, 2012 ), such as cognitive enhancement therapy (CET) for schizophrenia ( Hogarty et al., 2004 ) and autism ( Eack et al., 2013 ), and neuroplasticity-based computerized cognitive remediation for older adults with depression ( Morimoto et al., 2012 ). Participation in such interventions is thought to capitalize on neurobiological plasticity reserves to restore or enhance neural connections through repeated practice of cognitive exercises in an enriched social environment ( Keshavan & Hogarty, 1999 ; Morimoto et al., 2014 ). Unfortunately, outside of cognitive remediation, few discoveries surrounding brain plasticity have been translated to the development or refinement of other clinical interventions for mental illness ( Cramer et al., 2011 ), although notable examples are emerging ( Matto et al., 2013 ).
Overall, the neuroplasticity literature validates the long-held biopsychosocial perspective of social workers that socioenvironmental and genetic factors interact to influence neurobiology ( Garland & Howard, 2009 ). In the context of mental health treatment research, the principles of neuroplasticity are also reminiscent of the foundational framework of person-in-environment ( Bartlett, 1970 ) that postulates a bidirectional relationship between neurobiology and environment ( Green & McDermott, 2010 ). The dynamic, neuroplastic nature of the brain affords many opportunities for social workers to use cognitive neuroscience approaches in their translational research, thereby facilitating the development of more targeted and effective interventions that could directly address core pathophysiological processes to promote mental health recovery.
How Can Cognitive Neuroscience Research Improve Mental Health Treatment?
Early detection of people at risk for mental illness.
The current system for diagnosing mental health conditions is the Diagnostic and Statistical Manual of Mental Disorders, Fifth Edition (DSM-5; American Psychiatric Association, 2013 ). In this system, a diagnosis is made after the onset of mental illness based on presenting behavioral symptoms that meet a set of criteria. However, neural signs of mental health symptoms are often present years before the onset of the condition, opening the window for very early intervention or possible prevention. These neural signs are known as biomarkers —biological indicators of a medical condition or disease state. In the context of cognitive neuroscience research, a biomarker is a measurable neurobiological feature indicating that a person is at risk of developing certain mental health symptoms ( Singh & Rose, 2009 ). Abnormal patterns in brain activation while performing a task and/or reduced gray matter volume in regions supporting cognitive functioning are simple but frequently reported examples of neural biomarkers that may signal mental illness vulnerability ( Chang et al., 2017 ; Wackerhagen et al., 2017 ; Walter et al., 2016 ). Biomarkers are an ongoing area of investigation, and a valid and reliable marker for diagnosis does not yet exist for any major mental health condition. However, the use of neuroimaging-based biomarkers has the exciting potential to make mental health screening and diagnostic models more accurate, thereby increasing scientific opportunities for applied clinical research in very early intervention and preventive treatments.
Multivariate statistics—methods for analyzing multiple dependent variables at once (i.e., partial least squares)—are a common and robust approach to mental health biomarker research ( Haxby, Connolly, & Guntupalli, 2014 ). In the neuroscience literature, this statistical approach is generally known as multivariate pattern analysis or multivariate pattern recognition. Simply put, multivariate pattern analysis uses data on gray matter volume from MRI or brain activity from fMRI (the dependent variables) to classify people with and without a mental illness with high sensitivity and specificity ( Borgwardt & Fusar-Poli, 2012 ; Krishnan, Williams, McIntosh, & Abdi, 2011 ). Sensitivity indicates the ability of multivariate pattern analysis to correctly identify the proportion of individuals with a mental illness in a given sample, and specificity is the ability to correctly identify the proportion of individuals without a mental illness. For example, two recent meta-analyses were conducted across all neuroimaging studies using multivariate pattern recognition in major depression ( Kambeitz et al., 2016 ) and schizophrenia ( Kambeitz et al., 2015 ). Across 33 included neuroimaging studies, differential patterns in brain structure and activity correctly classified individuals with major depression and healthy volunteers at 77% sensitivity and 78% specificity. The meta-analytic findings among 38 neuroimaging studies in schizophrenia were similar, with high sensitivity (80%) and specificity (80%) in correctly classifying individuals with schizophrenia and healthy volunteers based on patterns in brain structure and function ( Kambeitz et al., 2015 ). Multivariate pattern analysis has also demonstrated capabilities in deciphering between different mental health diagnoses. Using resting-state fMRI data, multivariate pattern analysis correctly classified individuals with schizophrenia at 92% sensitivity and individuals with bipolar disorder at 83% sensitivity ( Calhoun, Maciejewski, Pearlson, & Kiehl, 2008 ). These findings have important implications for the use of neuroimaging biomarkers in diagnostic models that can accurately detect individuals very early in the course of illness to avoid long durations of untreated illness, inappropriate treatments, and disability.
From a more preventive perspective, multivariate pattern analysis has shown success in classifying individuals at ultra-high risk of developing psychosis. With a 92% sensitivity rate, structural MRI patterns differentiated individuals who developed psychosis from healthy volunteers ( Koutsouleris et al., 2012 ). The classification accuracy rate for the ultra-high risk individuals who did not transition to psychosis from those who did was 84% based on structural brain patterns. In trauma-related conditions, gray matter pattern differences were used to accurately classify 67% of trauma survivors who developed PTSD compared to trauma survivors who did not develop PTSD ( Gong et al., 2013 ). Such results have exciting implications for identifying and personalizing trauma-related interventions based on biomarkers that can predict those at risk of developing PTSD after a traumatic experience. Using diagnostic models from structural and functional brain scans has significant clinical utility for identifying phenotypes of mental illness risk and recovery. As such, the use of cognitive neuroscience research to detect neuroimaging-based biomarkers that are predictive of several forms of mental illness can improve mental health treatment by moving it from a tertiary form of prevention to a practice of primary prevention.
It should be noted, however, that there are several ethical concerns in neuroimaging-based biomarker research that are worthy of discussion ( Singh & Rose, 2009 ). First and foremost, before biomarker research can be translated to clinical practice, there must be a careful, cautious approach to developing therapeutic practice methods for when and how to inform people about their risks and/or resiliencies to mental illness based on neurobiology. This leads to the second ethical dilemma of biomarker research: that identifying a client at risk for mental illness can be potentially traumatizing and stigmatizing, especially considering that multivariate pattern analysis does not yet have 100% classification sensitivity. Third, biomarkers have only been studied in the context of research labs, making generalizability to clinical practice low ( Cook, 2008 ). Lastly, the use of biomarkers is costly, as MRI scanners are expensive and may not be accessible in rural communities. Trained experts are also required to operate the scanner, analyze the data, and interpret the findings ( Lakhan, Vieira, & Hamlat, 2010 ). Despite these considerations and the need for increased accuracy in multivariate models, cognitive neuroscience research aimed at identifying biomarkers of mental illness holds promise for improving mental health treatment by directing interventions to those who are most vulnerable. Pattern-recognition methods show particular promise for paving the way for effective early intervention strategies to prevent disability ( Woo, Chang, Lindquist, & Wager, 2017 ).
Identifying those who are resilient to mental illness
Neuroimaging-based biomarker research is also a rich area for identifying the brain signatures of resiliency. By understanding who is exposed to an adverse social environment and does not develop a mental health condition, we can learn about how the brain can protect itself. The study of the neural substrates that protect people from developing a mental health condition is a new area of investigation. However, themes are emerging suggesting that better regulated and organized neural networks for reward processing, emotion regulation, and stress management may be features of sustained mental health, even in the context of significant stress and trauma ( van der Werff, van den Berg, Pannekoek, Elzinga, & Van Der Wee, 2013 ). For example, a recent study by Swartz, Knodt, Radtke, and Hariri (2015) demonstrated that lower amygdala activation (implicated in emotion regulation) while viewing angry and fearful faces during a baseline scan in 340 healthy young adults predicted lower psychiatric vulnerability to life stressors up to 4 years later.
Neurobiological differences between people who develop or do not develop post-traumatic sequelae—such as PTSD ( Horn, Charney, & Feder, 2016 ) and major depression ( Han & Nestler, 2017 )—have provided additional clues for resiliency biomarkers of mental illness. Lower gray matter or reduced fear-related activation in the amygdala is a common protective biomarker of PTSD, as it may signal healthier regulation of emotional responses to stressful experiences ( Gupta et al., 2017 ; Kuo, Kaloupek, & Woodward, 2012 ; Morey, Haswell, Hooper, & De Bellis, 2016 ). Greater gray matter volume in the hippocampus and dorsolateral prefrontal cortex appear to be features of resiliency against developing major depression, especially for individuals with a family history of the condition ( Amico et al., 2011 ; Arnone, McIntosh, Ebmeier, Munafò, & Anderson, 2012 ; Rao et al., 2010 ). Explicit memory, which is the conscious retrieval of a thought, relies on communication (connectivity) between the dorsolateral prefrontal cortex and hippocampus and is a prominent cognitive challenge for people with major depression ( Pittenger & Duman, 2008 ). Therefore, having more and stronger neural connections from the dorsolateral prefrontal cortex to the hippocampus (e.g., larger gray matter volumes) underpinning effective explicit memory may be a neuroprotective factor against depression.
The neuro-resiliency field is growing, and common themes across mental health conditions have started to emerge. The amygdala has been prominently implicated in many studies, and this small limbic lobe brain structure is thought to be responsible, in part, for the generation of fear and other emotions ( Costafreda, Brammer, David, & Fu, 2008 ). A frequent finding is that an overactive amygdala places a person at greater risk for mental health challenges ( McLaughlin et al., 2014 ; Olsavsky et al., 2012 ; Zhong et al., 2011 ). In contrast, those whose amygdala appears to be under better control during stress are often less likely to experience trauma and other mental health-related symptoms ( Swartz et al., 2015 ). At the same time, increased integrity of prefrontal structures, which are involved in regulating amygdalar activity, also portend increased resiliency, even in the face of significant social and environmental adversity that would often contribute to the onset of a mental health condition ( Herringa et al., 2016 ; Tottenham & Galván, 2016 ). Such findings highlight the complex interplay between brain networks in supporting healthy psychological adjustment and begin to indicate the optimal states of the brain that can support resiliency and adjustment during the experience of life stressors.
Having a greater number of whole-brain neuronal connections (known as “brain reserve”) is another promising neural marker for resiliency ( Satz, 1993 ; Stern, 2002 ). Historically, the protective effects of brain reserve were discovered in postmortem brain studies of Alzheimer’s disease. Neuroscientists observed that, despite the presence of neuropathic plaques and tangles of Alzheimer’s disease in the postmortem brains, certain individuals did not experience the cognitive signs or symptoms of the disease as they aged ( Katzman et al., 1988 ). Such findings indicate that pathophysiology can exist in the brain, but protective mechanisms such as brain reserve can intervene in the clinical manifestation of the condition ( Mortimer, 1997 ). According to Mortimer (1997) , the brain reserve mechanism of action is thought to be a redundancy or larger number of neural connections that are readily available to compensate for the occurrence of lesions or disease insult to the brain ( Keshavan et al., 2011 ).
To summarize, neuroimaging-based biomarkers of resiliency have obvious clinical utility for screening, prevention, and personalization of mental health treatment through an understanding of how the brain can protect itself. Neuro-resiliency research will aid in the identification of which neural circuits must be strengthened to prevent mental illness and promote healthy psychosocial functioning and adjustment. In the social work context, neuro-resiliency research also raises new person-in-environment questions around why some people do not acquire neurodevelopmental impairments or harm in the face of extreme stress. For example, given the malleable nature of the brain, do resilient individuals who experience adversity, oppression, or discrimination have a more neuroplastic brain that is capable of rapidly adapting to change in the social environment, compared to less resilient people? How can psychosocial interventions facilitate a neurobiological foundation that is resilient to stress in some of the most underprivileged and oppressed populations?
Identifying neural treatment targets for mental health interventions
Cognitive neuroscience has perhaps gained the most traction in promoting mental health treatment through the identification of neural targets for intervention. By understanding the neurobiological mechanisms of mental illness, cognitive neuroscience research has generated a significant amount of evidence indicating where to target medications, brain stimulation, and psychosocial interventions. By providing in vivo measurements of brain function and structure during mental health treatment, cognitive neuroscience provides a window to observe the relationship between treatment parameters and distinct clinical and functional improvements. Such research will increase opportunities to make mental health treatments more targeted and precise. For example, suppose a new cognitive training intervention was observed to increase prefrontal brain activity associated with improved attention and ability to maintain employment. This observation would indicate that it may be optimal to emphasize certain training exercises associated with prefrontal cognitive control and attention for functional recovery in the employment domain. Intervention developers can then use this information to modify their cognitive training program to focus on the attention-training elements to provide a more targeted, less lengthy treatment experience that could be increasingly efficacious for improving vocational functioning. This target-engagement approach is an essential component of experimental medicine (see Insel, 2012 , p. 24), an approach to developing mental health interventions adopted by NIMH ( Insel, 2015 ). Clearly, such knowledge would help intervention research to prioritize novel and refined treatment strategies to improve mental health interventions.
Cognition, daily functioning, and motivation are all behavioral domains that are frequently impacted by mental health conditions. The identification and understanding of the neural underpinnings driving these behavioral domains can serve as mental health treatment targets. For example, in people with schizophrenia, lower gray matter volume in frontal, temporal, and limbic regions is associated with challenges in social and nonsocial cognition ( Wolf, Höse, Frasch, Walter, & Vasic, 2008 ; Yamada et al., 2007 ). Meta-analytic evidence has similarly demonstrated that lower gray matter volume in frontal and limbic regions is a neural correlate of functional abilities in people with schizophrenia ( Wojtalik, Smith, Keshavan, & Eack, 2017 ). More specifically, better social functioning in people with schizophrenia is predicted by greater empathy-related activation in the middle cingulate cortex of the limbic lobe ( Smith et al., 2015 ) and increased connectivity between the medial prefrontal cortex and posterior cingulate cortex ( Fox et al., 2017 ). In people with schizophrenia, there is substantial regional overlap among the frontal, limbic, and temporal lobes, which support cognitive and functional outcomes. These brain regions may be important neurobiological substrates that could be targeted through pharmacological and/or psychosocial interventions to promote a more complete recovery from schizophrenia.
Regarding motivation, the striatum is an important limbic lobe region affecting motivational outcomes across people with schizophrenia, major depression, and bipolar disorder ( Whitton, Treadway, & Pizzagalli, 2015 ). This transdiagnostic neural target is associated with underactivation in people with major depression and schizophrenia, which translates to a lack of motivation (i.e., negative symptoms, anhedonia). In bipolar disorder, hyperactivation in the striatum is linked to oversensitivity to reward and high motivation for rewarding experiences (i.e., mania; Whitton et al., 2015 ). Compared to healthy volunteers, individuals with bipolar disorder recruit significantly more striatal activity during the anticipation of monetary rewards, possibly representing a neural determinant of elevated mood ( Nusslock et al., 2012 ). Others have observed lower striatal activation during reward processing in major depression and schizophrenia. For example, Subramaniam et al. (2015) observed significantly less activation in the striatum during monetary reward anticipation in people with schizophrenia when compared to healthy individuals. In healthy volunteers, greater activation in the striatum was associated with an increased sense of pleasure and motivation after receiving a monetary reward, but similar activation was not observed in individuals with schizophrenia.
For interventionists, these results suggest that increasing recruitment of the striatum during anticipatory reward processing in clients with major depression and schizophrenia (and decreasing striatal activity in bipolar disorder) is a potentially promising treatment target to improve motivational difficulties. For example, behavioral treatments that encourage increased motivation by positively reinforcing rewarding behaviors ( Favrod, Giuliani, Ernst, & Bonsack, 2010 ) such as exercising ( Dauwan, Begemann, Heringa, & Sommer, 2016 ; Firth, Cotter, Elliott, French, & Yung, 2015 ) appear to activate the striatum ( Robertson et al., 2016 ). Repeated reinforcement of a rewarding behavior may alter the striatum so that over time it will become more intrinsically activated by the anticipation of reward, which may generalize to improved motivational outcomes in clients outside of the clinic. In this way, knowledge of the neural contributors to different domains of behavioral outcomes in people with mental health conditions can improve the precision of mental health treatments by providing direct neurobiological targets for intervention. Based on growing data on the plasticity of the brain and its impact by the social environment, it is increasingly plausible to address these neural targets with non-pharmacological interventions ( Keshavan et al., 2014 ).
Using cognitive neuroscience research to personalize mental health treatment
An emerging theme in the earlier sections of this paper is the strong potential for biomarkers and treatment targets to help make mental health treatment more personalized. Because mental illness has a substantial brain component, and even single conditions are highly heterogeneous, understanding a person’s neurobiology will help select the treatment protocols most likely to work the first time, or most quickly, rather than having clients endure repeated treatment failures. The complex interplay between genetics, environment, and neurobiology—along with the uniqueness of each person—makes true personalization of mental health treatment quite challenging. However, neuroimaging-based biomarkers provide information about neurobiological features that could accurately determine which pharmacological or psychosocial treatments would be the best fit for specific clients, at least from a neurobiological perspective ( Holsboer, 2008 ). The NIMH promotes the utility of cognitive neuroscience tools for identifying neural substrates that can be used to personalize mental health treatment ( Cuthbert, 2014 ). The Research Domain Criteria (RDoC) is an NIMH initiative that encourages a dimensional approach to understanding mental illness as an alternative to the current categorical diagnostic system ( Insel et al., 2010 ; NIMH, n.d. ). The strategic goal of RDoC is to fund research that will identify the neural basis of differential dimensions of observable behavior and functioning in order to inform treatment personalization based on neurobiological features ( Morris & Cuthbert, 2012 ).
The use of cognitive neuroscience to predict responsiveness to treatment has been a particularly important avenue for determining the neural mechanisms of personalizing mental health treatment. For example, an early study by Bryant et al. (2008) observed that optimal response to cognitive–behavioral therapy (CBT) 6 months after completion was related to lower fear-related activity in the amygdala and anterior cingulate cortex of the limbic lobe in clients with PTSD. Lower amygdala reactivity to fearful faces is likely reflective of a lower fear response ( Bryant et al., 2008 ). A more recent study in PTSD found that having larger hippocampal volume at the start of prolonged exposure was predictive of better treatment response ( Rubin et al., 2016 ). The capacity to extinguish traumatic memories may be a capability associated with larger hippocampal volume ( Rubin et al., 2016 ). In the context of treatment personalization, it can be inferred that the absence of these neurobiological features (i.e., lower amygdala reactivity and larger hippocampus) in clients with PTSD is rate-limiting. These clients may require emotion regulation and memory training prior to the start of CBT or prolonged exposure to optimize response.
In the case of early course schizophrenia, having a greater amount of cortical gray matter at the start of cognitive remediation is associated with an accelerated improvement in social cognition. People with lower cortical gray matter benefit from treatment, but the rate of social cognitive improvement is slower ( Keshavan et al., 2011 ). Such results indicate that cortical gray matter volume can be assessed at the start of cognitive remediation to personalize the length of treatment and which cognitive domains to target for optimizing recovery. Finally, Dunlop et al. (2017) used multivariate pattern analysis to examine connectivity signatures of clients with major depression who responded to CBT and antidepressant medication compared to clients who were not responsive to treatment. Differential resting-state connectivity patterns in the cingulate cortex accurately classified clients who failed to respond to treatment against clients who did respond at an average of 82%. These results again demonstrate the clinical utility of using cognitive neuroscience to aid in the identification of effective first-line treatment. This is particularly important given that treatment failures and the revolving-door experience can be demoralizing for clients and a significant barrier to treatment engagement ( Andrade et al., 2014 ).
How Can Mental Health Treatment Identify Brain Mechanisms Underlying Cognition, Affect, and Behavior?
Although much can be learned about improving mental health treatment by incorporating data and discoveries from cognitive neuroscience, intervention research also provides a powerful platform for understanding the brain and how it changes when exposed to treatment (see Table 1 ). The longitudinal context of treatment research, as well as the experimental manipulation of interventions, goes far beyond the cross-sectional studies that characterize much of cognitive neuroscience. Clinical trials afford unique opportunities for understanding the plasticity of specific brain regions, how the brain changes in response to psychosocial intervention, and the connection between brain changes and meaningful treatment outcomes. The capacity for intervention research to yield significant findings for cognitive neuroscience has been particularly represented by the experimental-medicine approach adopted by NIMH ( Insel, 2015 ).
Experimental medicine
Experimental medicine is a novel approach adopted by NIMH to, in part, integrate biological and treatment research, and to use the experimental and longitudinal context of clinical trials to yield new cognitive neuroscience discoveries in a more robust context than cross-sectional research. The basic premise of the approach is that interventions to improve mental health usually have underlying targets that mediate treatment outcomes. More specifically, this approach uses neural markers and their change by treatments as proxy outcome measures in clinical trials, thereby making treatment research more efficient and leading to quicker answers ( Lewandowski, Ongur, & Keshavan, 2018 ). These targets often implicate cognitive neuroscience constructs, such as increased brain communication and better outcomes in autism ( Plitt, Barnes, Wallace, Kenworthy, & Martin, 2015 ) or lower prefrontal activity predicting social disability in schizophrenia ( Wojtalik et al., 2017 ). The experimental-medicine approach makes these intervention targets explicit foci of treatment. The experimental context of clinical trials is used to intervene on these targets and assess the impact on meaningful mental health outcomes ( Insel, 2012 ). It is hypothesized that two important advances will result from this approach. First, mental health treatment research will accelerate by focusing on target engagement and a “fast-fail” approach to intervention development. In such an approach, researchers perform smaller and more rapid clinical trials to identify treatment targets that show malleability and connection to favorable outcomes and quickly abandon those targets that do not ( Insel & Gogtay, 2014 ). Second, the underlying mechanisms contributing to mental illness will be discovered as researchers identify which targets contribute to mental health outcomes. As such, experimental medicine views treatment research not only as a tool for building better interventions, but also for discovering the targets—often neurobiological in nature—that do and do not contribute to mental health ( Insel, 2012 , 2015 ).
The experimental-medicine paradigm comes from the broader biomedical treatment literature for physical illnesses, including oncology. Experimental medicine is an attempt by NIMH and the scientific community to align mental health intervention research with medical research in other fields. Major advances in cancer research have been accomplished by increasing the focus on potential targets for tumor development and proliferation, ruling out those targets that show no signs of contributing to pathophysiology and noting targets that are less tractable to intervention ( Jones & Price, 2012 ). Many of the most significant treatment advances in mental health have serendipitously taken this route, such as when dopamine antagonists were discovered to treat schizophrenia and serotonin reuptake inhibitors were identified for the treatment of depression. The neural contributors to these conditions were largely formulated around observations that such medications affected a neural target, and when that neural target changed, mental health symptoms improved substantially. This is why schizophrenia is known to involve dopaminergic hyperactivity and why depression is known to involve serotonin neurotransmission. However, not all mental health issues are amenable to such an approach ( Markowitz, 2016 ). Experimental medicine is an attempt to learn more from treatment research—not only about what works, but about the mechanisms that contribute to and ultimately cause mental illness. As such, treatment research can significantly inform research on the cognitive neuroscience of mental health, particularly in understanding where and how the brain can change, as well as the changes that are most needed to support improved recovery and psychosocial functioning.
Identifying where the brain changes in response to mental health treatment
The discovery of brain plasticity and the significant interplay between the social environment and neural change has ushered in a new era of understanding the capabilities of human neurobiology. It is now widely recognized that the brain is malleable and continuously shaped beyond early development by learning, interaction, and the environment ( Bruel-Jungerman et al., 2007 ; Buonomano & Merzenich, 1998 ). What is less known is where the brain can change, and treatment research is well poised to contribute knowledge to this area. Like many phenomena, brain plasticity is expected to be unevenly distributed ( Buonomano & Merzenich, 1998 ). Perhaps the most “neuroplastic” area discovered to date is the hippocampus, a region in the middle of the brain that is heavily involved in memory storage. This finding is largely drawn from experimental animal studies ( Soya et al., 2007 ; Van Praag, Shubert, Zhao, & Gage, 2005 ), and human exercise-physiology studies ( Erickson et al., 2011 ; Fuss et al., 2014 ). Researchers have conducted numerous studies on the impact of aerobic activity on hippocampal volume and memory across many different population groups. For example, Erickson and colleagues (2011) completed a randomized trial of a 40-minute walking intervention versus a stretching control in older adults and found that hippocampal volume was significantly increased in the moderate exercise condition relative to control. Furthermore, these volumetric changes were associated with significant improvements in memory, perhaps reversing age-related memory decline by several years ( Erickson et al., 2011 ).
For some time it was thought that only the hippocampus might possess this remarkable ability to self-repair and generate new neurons ( Deng, Aimone, & Gage, 2010 ), but additional studies are emerging indicating that plasticity is possible in other areas of the brain to support improved function in different domains. For example, Wang and colleagues (2016) examined the effects of CBT on brain communication patterns in adults with attention deficit hyperactivity disorder and found significant increases in the communication between frontal and parietal regions among participants treated with CBT. These neural changes were associated with reduced symptoms of attention deficit hyperactivity disorder ( Wang et al., 2016 ). Another study of CBT by Shou and colleagues (2017) found increased frontoparietal connectivity with the amygdala in adults with stress-related conditions who were receiving treatment, again suggesting that CBT can impact neural communication patterns across diverse areas of the brain in support of improved mental health outcomes ( Shou et al., 2017 ). A recent meta-analytic review of the impact of cognitive remediation interventions on brain function in schizophrenia found widespread increases in brain activity throughout the prefrontal cortex associated with treatment ( Ramsay & MacDonald, 2015 ). Although these intervention studies focus on brain function and communication, and it remains unclear the degree to which the generation of new neurons outside of the hippocampus is possible, it is increasing evident that many brain regions have greater functional plasticity than previously recognized.
Much of what is known about the capacity of different regions of the human brain to change has been generated from mental health intervention research. Whether it is aerobic interventions to protect against memory loss or cognitive exercises to enhance attention and problem-solving, these studies are a driving force behind what is known about where the brain can change. The longitudinal and often experimental nature of mental health treatment research provides a powerful platform for probing and understanding brain plasticity. Although a great deal remains to be learned and the distribution of functional and structural plasticity throughout the human brain is only beginning to be mapped, there is considerable optimism that much of our neural make-up could be amenable to positive change and influence ( Cramer et al., 2011 ; Garland & Howard, 2009 ). Mental health treatment research will be an essential contributor to building knowledge in this area.
Discovering how the brain changes in response to mental health treatment
In addition to understanding where the brain can change, research on mental health interventions can also provide important information on how the brain changes. As the field is learning more about the impact of mental health interventions on different regions of the brain, new findings indicate that multiple avenues exist for improving mental health outcomes through neural change. A primary question for the field at this time is whether brain parameters need to be restored to those equivalent to healthy or unaffected individuals, or whether the brain might compensate and chart completely novel avenues of function and structure to improve outcomes ( Penadés et al., 2017 ). There is evidence from the stroke and rehabilitation literature that compensation may be a common way for the brain to improve function ( Murphy & Corbett, 2009 ). For example, a study by Small, Hlustik, Noll, Genovese, and Solodkin (2002) observed that participants who regained limb function after a motor-impacting stroke had significant increases in motor cortex activity in the hemisphere opposite of the affected area of the brain. This suggested neural compensation: that the brain was rerouting motor activity from the damaged hemisphere to the functional one to improve movement ( Small et al., 2002 ).
In the mental health field, evidence is emerging that both compensatory and restorative changes are possible. For example, in a study of cognitive remediation in people living with schizophrenia, Penadés and colleagues (2013) found that pre-frontal brain function became more normalized and similar to healthy individuals during the course of treatment, although the implications for behavior were less clear. Another study of neural feedback training in children with attention deficit hyperactivity disorder found that individuals could up-regulate a key region of the prefrontal cortex similar to healthy individuals, with significant improvements in hyperactivity and inattentive symptoms ( Alegria et al., 2017 ). Further, a study of age-related memory loss found that strategy-based memory training produced increased brain activity in novel regions not active prior to treatment, suggesting the compensatory recruitment of brain regions to enhance memory function in older adults ( Belleville et al., 2015 ). In addition, a study of children with abuse-related PTSD observed a normalization of frontotemporal activation during the course of CBT, which was associated with reduced emotional arousal; this indicated possible normalization of some aspects of brain function contributing to clinical recovery ( Thomaes et al., 2012 ).
These neuroimaging studies of mental health interventions provide important insights into how the brain changes to improve cognition, psychosocial functioning, and recovery. Although this area of research is in its infancy, evidence exists across a wide range of conditions for the possibility of both restorative and compensatory mechanisms involved in brain change associated with mental health improvement. Understanding whether a neuroimaging effect represents compensation or restoration is heavily dependent upon a firm grasp of neurotypical brain function and abnormalities associated with disorder. Both cognitive and clinical neuroscience are rapidly generating such data, and research from mental health interventions can help determine not only where the brain changes, but also whether normalization results from treatment or whether functional improvements can be gained through compensatory processes.
Linking treatment-related brain change to meaningful behavioral outcomes
From the discussion of how mental health research can inform cognitive neuroscience, it should be increasingly clear that the brain can change as a result of mental health intervention. However, not all changes are meaningful or helpful, and simply discovering that a region is amenable to change will do little to help those living with a disability if such changes are not linked to meaningful outcomes. The integration of mental health treatment research with cognitive neuroscience can reveal how treatment-related brain changes support real-world functioning and recovery, which is likely most pertinent to the underserved populations social workers serve in community mental health clinics.
The use of cognitive neuroscience techniques in mental health treatment research can demonstrate specific locations in the brain altered by intervention that subsequently had a positive influence on symptoms, cognition, and/or functioning. For example, individuals with major depression have difficulty processing emotional information. Such challenges reflect underactivation of frontolimbic regions, which Ritchey, Dolcos, Eddington, Strauman, and Cabeza (2011) demonstrated could be reversed by participating in CBT. After completing an average of 21 CBT sessions, individuals with major depression had significant pre- to posttreatment increases in brain activation in the ventromedial prefrontal cortex, amygdala, caudate, hippocampus, and the anterior temporal lobe while evaluating emotional faces in the scanner. However, only the ventromedial prefrontal cortex and anterior temporal lobe predicted meaningful improvement in depression symptomatology ( Ritchey et al., 2011 ). CBT is a comprehensive program that attempts to address several aspects of thinking (i.e., evaluating automatic thoughts, identifying feelings, and modifying beliefs). Therefore, such results indicate that although other brain areas were alerted by CBT, the ventromedial prefrontal cortex and anterior temporal lobe may have a meaningful link to emotional processing abilities and relieving symptoms of depression—a marked advance in understanding the functional significance of these brain changes.
In individuals with autism, difficulty recognizing emotions in others is a prominent feature of the condition ( Lozier, Vanmeter, & Marsh, 2014 ; Uljarevic & Hamilton, 2013 ). Reduced activation in the fusiform gyrus, a temporal lobe region implicated in facial-affect recognition, is a commonly observed neural correlate of social cognitive impairment in autism ( Corbett et al., 2009 ; Schultz, 2005 ). Bölte et al. (2006) hypothesized that after 5 weeks of facial-affect recognition training, individuals with autism would show significant behavioral improvement in facial-affect recognition associated with increased activity in the fusiform gyrus. However, increased activation in the superior parietal lobule and right medial occipital lobe, not the fusiform gyrus, were significantly correlated with improved behavioral facial-affect recognition skills after training ( Bölte et al., 2006 ). Similarly, a more recent study observed that improved interpersonal communication scores were significantly related to medial prefrontal cortex activation—rather than the assumed fusiform gyrus—following facial-affect recognition training ( Bölte et al., 2015 ). These results further demonstrate the importance of integrating neuroimaging measures into clinical trials to advance our understanding of where change in the brain matters for improving psychosocial functioning and recovery.
Repetitive transcranial magnetic stimulation (rTMS) is a noninvasive treatment that uses targeted brain stimulation by sending a small but strong electrical current into the cerebral cortex to alter brain functioning. rTMS has treated several mental health conditions ( Slotema, Dirk Blom, Hoek, & Sommer, 2010 ). Given that this treatment is a focused approach for stimulating selected brain regions with the goal to reduce behavioral symptoms, rTMS exemplifies the importance of linking brain change to meaningful outcomes. This treatment has been applied to the positive and negative symptoms of schizophrenia, albeit with mixed findings for efficacy ( Hasan et al., 2017 ; Kimura et al., 2016 ). rTMS treatments traditionally target the left dorsolateral prefrontal cortex to address negative symptoms and the left temporoparietal cortex to reduce positive symptoms in the condition ( Freitas, Fregni, & Pascual-Leone, 2009 ; Shi, Yu, Cheung, Shum, & Chan, 2014 ). Despite target engagement of these theorized regions by rTMS, there often is no observed effect on clinical outcome (i.e., reduced hallucinations). For example, in a double-blind, randomized controlled trial, Novak et al. (2006) applied rTMS to the left dorsolateral prefrontal cortex for 10 days and observed no significant improvements in negative symptom scores. The variability of efficacy findings in rTMS for schizophrenia likely reflects a lack of knowledge of where to target rTMS to optimize the effects on meaningful behavioral change. Such rTMS studies underscore the importance of knowing the exact brain regions that need to be altered by an intervention in order to observe tangible improvements in clinical and functional outcomes in clients.
To truly optimize mental health treatment protocols, however, neuroimaging-based research will need go a step further and examine the degree to which brain changes associated with treatment impact more distal, real-world functioning goals. Preliminary cognitive neuroscience findings indicate that there is a neural signal associated with better social functioning in society ( Wojtalik et al., 2017 ), including financial stability in old age ( Han et al., 2014 ), income level ( Hanson, Chandra, Wolfe, & Pollak, 2011 ), socioeconomic status ( Noble, Houston, Kan, & Sowell, 2012 ), and marriage ( Petrican, Rosenbaum, & Grady, 2015 ). Knowledge of the reciprocal link between changes in the brain and meaningful progress in the ability to hold a competitive job, finish college, and live independently has vital implications for maximizing and personalizing pharmacological, brain stimulation, and psychosocial interventions. All of these outcomes are prominent goals for most clients working with social workers and are pertinent to clients’ overall quality of life ( Eack & Newhill, 2007 ). Conclusively, examining treatment-related brain alterations and their mediating/moderating role in psychosocial recovery is an imperative direction for improving the specificity and precision of mental health treatments to have a real and meaningful influence on clients’ ability to achieve personal goals.
Summary and Conclusions
Mental health conditions are severely and persistently disabling, placing significant barriers on individuals’ ability to recover and achieve personal goals, such as maintaining competitive employment and living independently. Despite significant advances in mental health treatment in both pharmacological and psychosocial arenas, functional recovery from mental health conditions remains inadequate. Such limits in mental health treatment are evidence of the need for novel methods to improve interventions. Recent advances in cognitive neuroscience offer unique opportunities to improve the effectiveness of mental health treatment. This paper reviewed how incorporating cognitive neuroscience into clinical research conducted by social workers and allied mental health professions may improve mental health interventions and promote greater functional recovery ( National Institutes of Health, 2015 ).
We reviewed several developing avenues that demonstrate the clinical utility of combining cognitive neuroscience and mental health treatment research (see Table 1 ). Cognitive neuroscience can improve mental health treatments so they are more preventive, early intervening, and targeted at core pathophysiology. Neuroimaging-based biomarker and treatment-target research can indicate brain signatures of risk and resiliency for mental health conditions or symptoms. Findings from such research directs interventionists to optimal locations in the brain to prevent onset or reduce long-term disability by intervening early in the course of an illness. The goal of linking clients’ neurobiology to treatment protocols is to increase personalization and decrease the lengthy process of finding the right treatment. Additionally, mental health treatment research provides a powerful context for cognitive neuroscience research. The longitudinal nature of clinical trials using neuroimaging measures provides additional information about where , how , and what in the brain can be altered by treatment to promote the greatest opportunities for complete, client-defined recovery from mental illness. Such knowledge will support the field in prioritizing novel and refined treatment strategies to improve interventions for people burdened by some of the most disabling effects of mental illness.
Of course, the brain is not the ultimate solution for developing and optimizing mental health treatments. Readers should be aware of the cautions, limitations, and reductionist nature of cognitive neuroscience methodologies (for a full review see Satel & Lilenfeld, 2013 ). Current neuroimaging tools capture an oversimplified and reduced picture of the intricate complexities of the physiology of the human brain and the black box of the mind. For example, fMRI provides a correlational link between changes in blood flow (i.e., a lighted blob on an image) and a single cognitive ability, such as working memory or empathy. Neurobiological changes during a cognitive paradigm measured with cognitive neuroscience techniques are not a direct representation of what a person is truly thinking or feeling, and it would be premature for researchers to make such claims ( Satel & Lilienfeld, 2013 ). Neuroimaging methods are merely a new and increasingly available tool for social workers to use in their research to address the biopsychosocial contributors to mental health. Our goal with this article is to encourage equal distribution of importance across all three domains of the biopsychosocial framework. Social workers often use psychosocial approaches in mental health treatment, but biology also requires attention to facilitate a comprehensive, holistic view of client circumstances. The integration of social work and cognitive neuroscience has considerable potential to improve the lives of people suffering from mental health conditions by attending to biology, the social environment, and their reciprocal interplay.
The use of cognitive neuroscience to improve mental health treatment also contributes to several of the 12 Grand Challenges for Social Work ( American Academy of Social Work and Social Welfare, n.d. ), such as (a) harnessing technology for social good, (b) advancing long and productive lives, (c) eradicating social isolation, and (d) achieving equal opportunity and justice. For example, DeVylder (2016) proposed that social workers lead collaborative efforts to develop innovative psychosocial treatments to prevent the onset of psychosis aligned with the grand challenge to ensure healthy development for all youth. The application of cognitive neuroscience findings indicating brain areas linked to the conversion of psychosis ( Dazzan et al., 2011 ; Smieskova et al., 2010 ; Thermenos et al., 2016 ) directly informs the development of prevention treatments, is consistent with the biopsychosocial framework, and is essential to the success of this grand challenge.
The integration of cognitive neuroscience and mental health treatment research is an exciting and innovative opportunity for improving intervention effectiveness. However, implementing neuroimaging measures can be intimidating and overwhelming. Social workers may be surprised by the richness of resources and the feasibility of incorporating cognitive neuroscience research. A first step to integrate cognitive neuroscience and mental health treatment research is the inclusion of neuroimaging findings from clinical trials in social work courses, which is already becoming a practice in psychiatry ( Etkin & Cuthbert, 2014 ). For general teaching resources, see Egan, Neely-Barnes, and Combs-Orme (2011) ; Farmer (2008) ; Matto et al. (2014) ; and Shapiro and Applegate (2000) . Additionally, cognitive neuroscience findings can be used as a clinical platform to destigmatize diagnoses and symptoms by educating clients and their family members about the neurobiology of mental health conditions and the ability of treatments to change the brain for the better.
Regarding methods and analysis, software for processing and analyzing neuroimaging data is freely available, such as Statistical Parametric Mapping (SPM; http://www.fil.ion.ucl.ac.uk/spm/resources ) and FSL ( https://fsl.fmrib.ox.ac.uk/fsl/fslwiki ). SPM requires MATLAB to operate, but it provides an easy-to-use graphical user interface and a detailed, step-by-step manual ( http://www.fil.ion.ucl.ac.uk/spm/doc/ ) with downloadable practice data across several neuroimaging modalities ( http://www.fil.ion.ucl.ac.uk/spm/data/ ). Statistical Analysis of fMRI Data ( Ashby, 2011 ) is a helpful, low-cost textbook that provides a straightforward and easy-to-read foundation for neuroimaging methods and analysis. Cognitive neuroscience training courses also are available for scientists without a traditional neuroscience background (see, for example, Neurometrika at http://neurometrika.org/ ).
Overall, by summarizing the cognitive neuroscience literature related to mental health treatment and providing resources, we hope that social workers and allied professions feel encouraged and capable of beginning to include cognitive neuroscience measures in their intervention studies. The next generation of mental health treatment advances will require such an integration, and a true biopsychosocial understanding of client strengths, challenges, and opportunities for intervention. The integration of cognitive neuroscience and mental health treatment research has the potential to eradicate lifelong disability by increasing the precision of client-driven, first-line treatment decisions.
Acknowledgments
This research was supported by National Institutes of Health grants MH 92440 (Matcheri S. Keshavan and Shaun M. Eack, principal investigators), MH 106450 (Shaun M. Eack, principal investigator), and MH 113277 (Jessica A. Wojtalik, principal investigator).
Contributor Information
Jessica A. Wojtalik, Doctoral candidate at the University of Pittsburgh School of Social Work.
Shaun M. Eack, Professor at the University of Pittsburgh School of Social Work and Department of Psychiatry.
Matthew J. Smith, Associate professor at the University of Michigan School of Social Work.
Matcheri S. Keshavan, Professor in the Harvard Medical School Department of Psychiatry.
- Adolphs R. The neurobiology of social cognition. Current Opinion in Neurobiology. 2001; 11 (2):231–239. https://doi.org/10.1016/S0959-4388(00)00202-6 [ PubMed ] [ Google Scholar ]
- Alegria AA, Wulff M, Brinson H, Barker GJ, Norman LJ, Brandeis D, Giampietro V. Real-time fMRI neurofeedback in adolescents with attention deficit hyperactivity disorder. Human Brain Mapping. 2017; 38 (6):3190–3209. doi: 10.1002/hbm.23584. [ PMC free article ] [ PubMed ] [ CrossRef ] [ Google Scholar ]
- American Academy of Social Work and Social Welfare. 12 challenges [web page] (n.d.) Retrieved from http://aaswsw.org/grand-challenges-initiative/12-challenges/
- American Psychiatric Association. Diagnostic and statistical manual of mental disorders. 5th. Washington, DC: Author; 2013. [ Google Scholar ]
- Amico F, Meisenzahl E, Koutsouleris N, Reiser M, Möller HJ, Frodl T. Structural MRI correlates for vulnerability and resilience to major depressive disorder. Journal of Psychiatry & Neuroscience: JPN. 2011; 36 (1):15–22. doi: 10.1503/jpn.090186. [ PMC free article ] [ PubMed ] [ CrossRef ] [ Google Scholar ]
- Anderson C, Reiss D, Hogarty GE. Schizophrenia and the family. New York, NY: Guilford Press; 1986. [ Google Scholar ]
- Andrade LH, Alonso J, Mneimneh Z, Wells JE, Al-Hamzawi A, Borges G, Kessler RC. Barriers to mental health treatment: Results from the WHO world mental health (WMH) surveys. Psychological Medicine. 2014; 44 (6):1303–1317. doi: 10.1017/S0033291713001943. [ PMC free article ] [ PubMed ] [ CrossRef ] [ Google Scholar ]
- Ansorge MS, Hen R, Gingrich JA. Neurodevelopmental origins of depressive disorders. Current Opinion in Pharmacology. 2007; 7 (1):8–17. https://doi.org/10.1016/j.coph.2006.11.006 [ PubMed ] [ Google Scholar ]
- Arnone D, McIntosh AM, Ebmeier KP, Munafò MR, Anderson IM. Magnetic resonance imaging studies in unipolar depression: Systematic review and meta-regression analyses. European Neuropsychopharmacology. 2012; 22 (1):1–16. doi: 10.1016/j.euroneuro.2011.05.003. [ PubMed ] [ CrossRef ] [ Google Scholar ]
- Ashby FG. Statistical analysis of fMRI data. Cambridge, MA: The MIT Press; 2011. [ Google Scholar ]
- Barch DM, Burgess GC, Harms MP, Petersen SE, Schlaggar BL, Corbetta M, Van Essen DC. Function in the human connectome: Task-fMRI and individual differences in behavior. NeuroImage. 2013; 80 :169–189. doi: 10.1016/j.neuroimage.2013.05.033. [ PMC free article ] [ PubMed ] [ CrossRef ] [ Google Scholar ]
- Barsaglini A, Sartori G, Benetti S, Pettersson-Yeo W, Mechelli A. The effects of psychotherapy on brain function: A systematic and critical review. Progress in Neurobiology. 2014; 114 :1–14. https://doi.org/10.1016/j.pneurobio.2013.10.006 [ PubMed ] [ Google Scholar ]
- Bartlett H. The common base of social work practice. New York, NY: National Association of Social Workers; 1970. [ Google Scholar ]
- Belleville S, Boller B, Ouellet É, Lecavalier NC, Mellah S, Gauthier S. Strategy-based memory training in persons with subjective cognitive decline: Efficacy, neural effects, and implications for models of training-induced compensatory plasticity. Alzheimer’s & Dementia: The Journal of the Alzheimer’s Association. 2015; 11 (7):P248. https://doi.org/10.1016/j.jalz.2015.07.307 [ Google Scholar ]
- Berger JM, Rohn TT, Oxford JT. Autism as the early closure of a neuroplastic critical period normally seen in adolescence. Biological Systems Open Access. 2013; 2 (3):1–7. doi: 10.4172/2329-6577.1000118. [ PMC free article ] [ PubMed ] [ CrossRef ] [ Google Scholar ]
- Bishop-Fitzpatrick L, Minshew NJ, Eack SM. A systematic review of psychosocial interventions for adults with autism spectrum disorders. In: Volkmar FR, Reichow B, McPartland J, editors. Adolescents and adults with autism spectrum disorders. New York, NY: Springer; 2014. pp. 315–327. [ PMC free article ] [ PubMed ] [ Google Scholar ]
- Bölte S, Ciaramidaro A, Schlitt S, Hainz D, Kliemann D, Beyer A, Walter H. Training-induced plasticity of the social brain in autism spectrum disorder. The British Journal of Psychiatry. 2015; 207 (2):149–157. doi: 10.1192/bjp.bp.113.143784. [ PubMed ] [ CrossRef ] [ Google Scholar ]
- Bölte S, Hubl D, Feineis-Matthews S, Prvulovic D, Dierks T, Poustka F. Facial affect recognition training in autism: Can we animate the fusiform gyrus? Behavioral Neuroscience. 2006; 120 (1):211–216. http://dx.doi.org/10.1037/0735-7044.120.1.211 [ PubMed ] [ Google Scholar ]
- Borgwardt S, Fusar-Poli P. Third-generation neuroimaging in early schizophrenia: Translating research evidence into clinical utility. The British Journal of Psychiatry. 2012; 200 (4):270–272. doi: 10.1192/bjp.bp.111.103234. [ PubMed ] [ CrossRef ] [ Google Scholar ]
- Brekke JS, Ell K, Palinkas LA. Translational science at the National Institute of Mental Health: Can social work take its rightful place? Research on Social Work Practice. 2007; 17 (1):123–133. https://doi.org/10.1177/1049731506293693 [ Google Scholar ]
- Bruel-Jungerman E, Davis S, Laroche S. Brain plasticity mechanisms and memory: A party of four. The Neuroscientist. 2007; 13 (5):492–505. https://doi.org/10.1177/1073858407302725 [ PubMed ] [ Google Scholar ]
- Bryant RA, Felmingham K, Kemp A, Das P, Hughes G, Peduto A, Williams L. Amygdala and ventral anterior cingulate activation predicts treatment response to cognitive behaviour therapy for post-traumatic stress disorder. Psychological Medicine. 2008; 38 (4):555–561. doi: 10.1017/s0033291707002231. [ PubMed ] [ CrossRef ] [ Google Scholar ]
- Buonomano DV, Merzenich MM. Cortical plasticity: From synapses to maps. Annual Review of Neuroscience. 1998; 21 (1):149–186. https://doi.org/10.1146/annurev.neuro.21.1.149 [ PubMed ] [ Google Scholar ]
- Bush G. Decade of the brain 1990–1999. Washington, DC: Government Publishing Office; 1990. Jul 17, (Proclamation No 6158 Stat 104). https://www.gpo.gov/fdsys/pkg/STATUTE-104/pdf/STATUTE-104-Pg5324.pdf . [ Google Scholar ]
- Calhoun VD, Maciejewski PK, Pearlson GD, Kiehl KA. Temporal lobe and “default” hemodynamic brain modes discriminate between schizophrenia and bipolar disorder. Human Brain Mapping. 2008; 29 (11):1265–1275. doi: 10.1002/hbm.20463. [ PMC free article ] [ PubMed ] [ CrossRef ] [ Google Scholar ]
- Cannon TD, Chung Y, He G, Sun D, Jacobson A, Van Erp TG, Cadenhead K. Progressive reduction in cortical thickness as psychosis develops: A multisite longitudinal neuroimaging study of youth at elevated clinical risk. Biological Psychiatry. 2015; 77 (2):147–157. https://doi.org/10.1016/j.biopsych.2014.05.023 [ PMC free article ] [ PubMed ] [ Google Scholar ]
- Chang K, Garrett A, Kelley R, Howe M, Sanders EM, Acquaye T, Reiss A. Anomalous prefrontal-limbic activation and connectivity in youth at high-risk for bipolar disorder. Journal of Affective Disorders. 2017; 222 :7–13. https://doi.org/10.1016/j.jad.2017.05.051 [ PubMed ] [ Google Scholar ]
- Charney DS, Buxbaum JD, Sklar P, Nestler EJ, editors. Neurobiology of mental illness. New York, NY: Oxford University Press; 2013. [ Google Scholar ]
- Chayer C, Freedman M. Frontal lobe functions. Current Neurology and Neuroscience Reports. 2001; 1 (6):547–552. https://doi.org/10.1007/s11910-001-0060-4 [ PubMed ] [ Google Scholar ]
- Cook IA. Biomarkers in psychiatry: Potentials, pitfalls, and pragmatics. Primary Psychiatry. 2008; 15 (3):54–59. [ Google Scholar ]
- Corbett BA, Carmean V, Ravizza S, Wendelken C, Henry ML, Carter C, Rivera SM. A functional and structural study of emotion and face processing in children with autism. Psychiatry Research. 2009; 173 (3):196–205. doi: 10.1016/j.pscychresns.2008.08.005. [ PMC free article ] [ PubMed ] [ CrossRef ] [ Google Scholar ]
- Costafreda SG, Brammer MJ, David AS, Fu CHY. Predictors of amygdala activation during the processing of emotional stimuli: A meta-analysis of 385 PET and f MRI studies. Brain Research Reviews. 2008; 58 (1):57–70. http://dx.doi.org/10.1016/j.brainresrev.2007.10.012 [ PubMed ] [ Google Scholar ]
- Craig AD, Craig A. How do you feel—now? The anterior insula and human awareness. Nature Reviews Neuroscience. 2009; 10 (1):59–70. doi: 10.1038/nrn2555. [ PubMed ] [ CrossRef ] [ Google Scholar ]
- Cramer SC, Sur M, Dobkin BH, O’Brien C, Sanger TD, Trojanowski JQ, Chen D. Harnessing neuroplasticity for clinical applications. Brain. 2011; 134 (6):1591–1609. https://doi.org/10.1093/brain/awr039 [ PMC free article ] [ PubMed ] [ Google Scholar ]
- Cuthbert BN. The RDoC framework: Facilitating transition from ICD/DSM to dimensional approaches that integrate neuroscience and psychopathology. World Psychiatry. 2014; 13 (1):28–35. doi: 10.1002/wps.20087. [ PMC free article ] [ PubMed ] [ CrossRef ] [ Google Scholar ]
- Dauwan M, Begemann MJH, Heringa SM, Sommer IE. Exercise improves clinical symptoms, quality of life, global functioning, and depression in schizophrenia: A systematic review and meta-analysis. Schizophrenia Bulletin. 2016; 42 (3):588–599. doi: 10.1093/schbul/sbv164. [ PMC free article ] [ PubMed ] [ CrossRef ] [ Google Scholar ]
- Davidson RJ, McEwen BS. Social influences on neuroplasticity: Stress and interventions to promote well-being. Nature Neuroscience. 2012; 15 (5):689–695. doi: 10.1038/nn.3093. [ PMC free article ] [ PubMed ] [ CrossRef ] [ Google Scholar ]
- Dazzan P, Soulsby B, Mechelli A, Wood SJ, Velakoulis D, Phillips LJ, Murray RM. Volumetric abnormalities predating the onset of schizophrenia and affective psychoses: An MRI study in subjects at ultrahigh risk of psychosis. Schizophrenia Bulletin. 2011; 38 (5):1083–1091. https://doi.org/10.1093/schbul/sbr035 [ PMC free article ] [ PubMed ] [ Google Scholar ]
- Dehaene S, Piazza M, Pinel P, Cohen L. Three parietal circuits for number processing. Cognitive Neuropsychology. 2003; 20 (3–6):487–506. https://doi.org/10.1080/02643290244000239 [ PubMed ] [ Google Scholar ]
- Deng W, Aimone JB, Gage FH. New neurons and new memories: How does adult hippocampal neurogenesis affect learning and memory? Nature Reviews Neuroscience. 2010; 11 (5):339–350. http://www.nature.com/nrn/journal/v11/n5/suppinfo/nrn2822_S1.html . [ PMC free article ] [ PubMed ] [ Google Scholar ]
- DeVylder JE. Preventing schizophrenia and severe mental illness: A grand challenge for social work. Research on Social Work Practice. 2016; 26 (4):449–459. https://doi.org/10.1177/1049731515622687 [ Google Scholar ]
- Diamond MC, Krech D, Rosenzweig MR. The effects of an enriched environment on the histology of the rat cerebral cortex. The Journal of Comparative Neurology. 1964; 123 (1):111–119. doi: 10.1002/cne.901230110. [ PubMed ] [ CrossRef ] [ Google Scholar ]
- Draganski B, Gaser C, Busch V, Schuierer G, Bogdahn U, May A. Neuroplasticity: Changes in grey matter induced by training. Nature. 2004; 427 :311–312. doi: 10.1038/427311a. [ PubMed ] [ CrossRef ] [ Google Scholar ]
- Driemeyer J, Boyke J, Gaser C, Büchel C, May A. Changes in gray matter induced by learning—revisited. PLoS ONE. 2008; 3 (7):e2669. https://doi.org/10.1371/journal.pone.0002669 [ PMC free article ] [ PubMed ] [ Google Scholar ]
- Dunlop BW, Rajendra JK, Craighead WE, Kelley ME, McGrath CL, Choi KS, Mayberg HS. Functional connectivity of the subcallosal cingulate cortex and differential outcomes to treatment with cognitive-behavioral therapy or antidepressant medication for major depressive disorder. American Journal of Psychiatry. 2017; 174 (6):533–545. https://doi.org/10.1176/appi.ajp.2016.16050518 [ PMC free article ] [ PubMed ] [ Google Scholar ]
- Eack SM, Greenwald DP, Hogarty SS, Bahorik AL, Litschge MY, Mazefsky CA, Minshew NJ. Cognitive enhancement therapy for adults with autism spectrum disorder: Results of an 18-month feasibility study. Journal of Autism and Developmental Disorders. 2013; 43 (12):2866–2877. doi: 10.1007/s10803-013-1834-7. [ PMC free article ] [ PubMed ] [ CrossRef ] [ Google Scholar ]
- Eack SM, Hogarty GE, Cho RY, Prasad KM, Greenwald DP, Hogarty SS, Keshavan MS. Neuroprotective effects of cognitive enhancement therapy against gray matter loss in early schizophrenia: Results from a 2-year randomized controlled trial. Archives of General Psychiatry. 2010; 67 (7):674–682. doi: 10.1001/archgenpsychiatry.2010.63. [ PMC free article ] [ PubMed ] [ CrossRef ] [ Google Scholar ]
- Eack SM, Newhill CE. Psychiatric symptoms and quality of life in schizophrenia: A meta-analysis. Schizophrenia Bulletin. 2007; 33 (5):1225–1237. https://doi.org/10.1093/schbul/sbl071 [ PMC free article ] [ PubMed ] [ Google Scholar ]
- Eack SM, Newhill CE, Keshavan MS. Cognitive enhancement therapy improves resting-state functional connectivity in early course schizophrenia. Journal of the Society for Social Work and Research. 2016; 7 (2):211–230. https://doi.org/10.1086/686538 [ PMC free article ] [ PubMed ] [ Google Scholar ]
- Egan M, Neely-Barnes SL, Combs-Orme T. Integrating neuroscience knowledge into social work education: A case-based approach. Journal of Social Work Education. 2011; 47 (2):269–282. https://doi.org/10.5175/JSWE.2011.200900109 [ Google Scholar ]
- Erickson KI, Voss MW, Prakash RS, Basak C, Szabo A, Chaddock L, White SM. Exercise training increases size of hippocampus and improves memory. Proceedings of the National Academy of Sciences. 2011; 108 (7):3017–3022. https://doi.org/10.1073/pnas.1015950108 [ PMC free article ] [ PubMed ] [ Google Scholar ]
- Etkin A, Cuthbert B. Beyond the DSM: Development of a transdiagnostic psychiatric neuroscience course. Academic Psychiatry. 2014; 38 (2):145–150. doi: 10.1007/s40596-013-0032-4. [ PubMed ] [ CrossRef ] [ Google Scholar ]
- Faludi G, Mirnics K. Synaptic changes in the brain of subjects with schizophrenia. International Journal of Developmental Neuroscience. 2011; 29 (3):305–309. https://doi.org/10.1016/j.ijdevneu.2011.02.013 [ PMC free article ] [ PubMed ] [ Google Scholar ]
- Farmer RL. Neuroscience and social work practice: The missing link. Thousand Oaks, CA: SAGE Publications; 2008. [ Google Scholar ]
- Favrod J, Giuliani F, Ernst F, Bonsack C. Anticipatory pleasure skills training: A new intervention to reduce anhedonia in schizophrenia. Perspectives in Psychiatric Care. 2010; 46 (3):171–181. doi: 10.1111/j.1744-6163.2010.00255.x. [ PubMed ] [ CrossRef ] [ Google Scholar ]
- Firth J, Cotter J, Elliott R, French P, Yung AR. A systematic review and meta-analysis of exercise interventions in schizophrenia patients. Psychological Medicine. 2015; 45 (7):1343–1361. doi: 10.1017/s0033291714003110. [ PubMed ] [ CrossRef ] [ Google Scholar ]
- Fischbach GD. Mind and brain. Scientific American. 1992; 267 (3):48–57. http://www.jstor.org/stable/24939212 . [ PubMed ] [ Google Scholar ]
- Fogassi L, Luppino G. Motor functions of the parietal lobe. Current Opinion in Neurobiology. 2005; 15 (6):626–631. https://doi.org/10.1016/j.conb.2005.10.015 [ PubMed ] [ Google Scholar ]
- Fossati P, Radtchenko A, Boyer P. Neuroplasticity: From MRI to depressive symptoms. European Neuropsychopharmacology. 2004; 14 :S503–S510. https://doi.org/10.1016/j.euroneuro.2004.09.001 [ PubMed ] [ Google Scholar ]
- Fox JM, Abram SV, Reilly JL, Eack S, Goldman MB, Csernansky JG, Smith MJ. Default mode functional connectivity is associated with social functioning in schizophrenia. Journal of Abnormal Psychology. 2017; 126 (4):392–405. doi: 10.1037/abn0000253. [ PMC free article ] [ PubMed ] [ CrossRef ] [ Google Scholar ]
- Frackowiak RS, editor. Human brain function. San Diego, CA: Academic Press; 2004. [ Google Scholar ]
- Freitas C, Fregni F, Pascual-Leone A. Meta-analysis of the effects of repetitive transcranial magnetic stimulation (rTMS) on negative and positive symptoms in schizophrenia. Schizophrenia Research. 2009; 108 (1):11–24. https://doi.org/10.1016/j.schres.2008.11.027 [ PMC free article ] [ PubMed ] [ Google Scholar ]
- Friston KJ. Functional and effective connectivity in neuroimaging: A synthesis. Human Brain Mapping. 1994; 2 :56–78. doi: 10.1002/hbm.460020107. [ CrossRef ] [ Google Scholar ]
- Friston KJ. Functional and effective connectivity: A review. Brain Connectivity. 2011; 1 (1):13–36. https://doi.org/10.1089/brain.2011.0008 [ PubMed ] [ Google Scholar ]
- Fuchs E, Flügge G. Adult neuroplasticity: More than 40 years of research. Neural Plasticity. 2014; 2014 :1–10. http://dx.doi.org/10.1155/2014/541870 [ PMC free article ] [ PubMed ] [ Google Scholar ]
- Fusar-Poli P, Radua J, McGuire P, Borgwardt S. Neuroanatomical maps of psychosis onset: Voxel-wise meta-analysis of antipsychotic-naive VBM studies. Schizophrenia Bulletin. 2011; 33 (6):1297–1307. doi: 10.1093/schbul/sbr134. [ PMC free article ] [ PubMed ] [ CrossRef ] [ Google Scholar ]
- Fuss J, Biedermann SV, Falfán-Melgoza C, Auer MK, Zheng L, Steinle J, Gass P. Exercise boosts hippocampal volume by preventing early age-related gray matter loss. Hippocampus. 2014; 24 (2):131–134. doi: 10.1002/hipo.22227. [ PubMed ] [ CrossRef ] [ Google Scholar ]
- Garland EL. Mindfulness-Oriented Recovery Enhancement for addiction, stress, and pain. Washington, DC: NASW Press; 2013. [ Google Scholar ]
- Garland EL, Froeliger B, Howard MO. Neurophysiological evidence for remediation of reward processing deficits in chronic pain and opioid misuse following treatment with Mindfulness-Oriented Recovery Enhancement: Exploratory ERP findings from a pilot RC T. Journal of Behavioral Medicine. 2015; 38 (2):327–336. https://doi.org/10.1007/s10865-014-9607-0 [ PMC free article ] [ PubMed ] [ Google Scholar ]
- Garland EL, Howard MO. Neuroplasticity, psychosocial genomics, and the biopsychosocial paradigm in the 21st century. Health & Social Work. 2009; 34 (3):191–199. https://doi.org/10.1093/hsw/34.3.191 [ PMC free article ] [ PubMed ] [ Google Scholar ]
- Goldberg TE, Hyde TM, Kleinman JE, Weinberger DR. Course of schizophrenia: Neuropsychological evidence for a static encephalopathy. Schizophrenia Bulletin. 1993; 19 (4):797–804. http://dx.doi.org/10.1093/schbul/19.4.797 [ PubMed ] [ Google Scholar ]
- Goldstein M. The decade of the brain. Neurology. 1990; 40 (2):321–321. http://dx.doi.org/10.1212/WNL.40.2.321 [ PubMed ] [ Google Scholar ]
- Gong Q, Li L, Tognin S, Wu Q, Pettersson-Yeo W, Lui S, Mechelli A. Using structural neuroanatomy to identify trauma survivors with and without post-traumatic stress disorder at the individual level. Psychological Medicine. 2013; 44 (1):195–203. doi: 10.1017/S0033291713000561. [ PMC free article ] [ PubMed ] [ CrossRef ] [ Google Scholar ]
- Goodkind M, Eickhoff SB, Oathes DJ, Jiang Y, Chang A, Jones-Hagata LB, Etkin A. Identification of a common neurobiological substrate for mental illness. JAMA Psychiatry. 2015; 72 (4):305–315. doi: 10.1001/jamapsychiatry.2014.2206. [ PMC free article ] [ PubMed ] [ CrossRef ] [ Google Scholar ]
- Green D, McDermott F. Social work from inside and between complex systems: Perspectives on person-in-environment for today’s social work. British Journal of Social Work. 2010; 40 (8):2414–2430. doi: 10.1093/bjsw/bcq056. [ CrossRef ] [ Google Scholar ]
- Gupta A, Love A, Kilpatrick LA, Labus JS, Bhatt R, Chang L, Mayer EA. Morphological brain measures of cortico-limbic inhibition related to resilience. Journal of Neuroscience Research. 2017; 95 (9):1760–1775. doi: 10.1002/jnr.24007. [ PMC free article ] [ PubMed ] [ CrossRef ] [ Google Scholar ]
- Han MH, Nestler EJ. Neural substrates of depression and resilience. Neurotherapeutics. 2017; 14 (3):677–686. doi: 10.1007/s13311-017-0527-x. [ PMC free article ] [ PubMed ] [ CrossRef ] [ Google Scholar ]
- Han SD, Boyle PA, Yu L, Fleischman DA, Arfanakis K, Leurgans S, Bennett DA. Financial literacy is associated with medial brain region functional connectivity in old age. Archives of Gerontology and Geriatrics. 2014; 59 (2):429–438. https://doi.org/10.1016/j.archger.2014.05.001 [ PMC free article ] [ PubMed ] [ Google Scholar ]
- Hanson JL, Chandra A, Wolfe BL, Pollak SD. Association between income and the hippocampus. PLoS ONE. 2011; 6 (5):e18712. https://doi.org/10.1371/journal.pone.0018712 [ PMC free article ] [ PubMed ] [ Google Scholar ]
- Harvey PD, Bellack AS. Toward a terminology for functional recovery in schizophrenia: Is functional remission a viable concept? Schizophrenia Bulletin. 2009; 35 (2):300–306. doi: 10.1093/schbul/sbn171. [ PMC free article ] [ PubMed ] [ CrossRef ] [ Google Scholar ]
- Hasan A, Wobrock T, Guse B, Langguth B, Landgrebe M, Eichhammer P, Koutsouleris N. Structural brain changes are associated with response of negative symptoms to prefrontal repetitive transcranial magnetic stimulation in patients with schizophrenia. Molecular Psychiatry. 2017; 22 (6):857–864. doi: 10.1038/mp.2016.161. [ PubMed ] [ CrossRef ] [ Google Scholar ]
- Haxby JV, Connolly AC, Guntupalli JS. Decoding neural representational spaces using multivariate pattern analysis. Annual Review of Neuroscience. 2014; 37 :435–456. https://doi.org/10.1146/annurev-neuro-062012-170325 [ PubMed ] [ Google Scholar ]
- Heisler EJ, Bagalman E. The mental health workforce: A primer (CRS Report No R43255) Washington, DC: Congressional Research Service; 2015. Retrieved from https://fas.org/sgp/crs/misc/R43255.pdf . [ Google Scholar ]
- Hermundstad AM, Bassett DS, Brown KS, Aminoff EM, Clewett D, Freeman S, Miller MB. Structural foundations of resting-state and task-based functional connectivity in the human brain. Proceedings of the National Academy of Sciences. 2013; 110 (15):6169–6174. https://doi.org/10.1073/pnas.1219562110 [ PMC free article ] [ PubMed ] [ Google Scholar ]
- Herringa RJ, Burghy CA, Stodola DE, Fox ME, Davidson RJ, Essex MJ. Enhanced prefrontal-amygdala connectivity following childhood adversity as a protective mechanism against internalizing in adolescence. Biological Psychiatry: Cognitive Neuroscience and Neuroimaging. 2016; 1 (4):326–334. doi: 10.1016/j.bpsc.2016.03.003. [ PMC free article ] [ PubMed ] [ CrossRef ] [ Google Scholar ]
- Hogarty GE, Flesher S, Ulrich R, Carter M, Greenwald D, Pogue-Geile M, Garrett A. Cognitive enhancement therapy for schizophrenia: Effects of a 2-year randomized trial on cognition and behavior. Archives of General Psychiatry. 2004; 61 (9):866–876. doi: 10.1001/archpsyc.61.9.866. [ PubMed ] [ CrossRef ] [ Google Scholar ]
- Holsboer F. How can we realize the promise of personalized antidepressant medicines? Nature Reviews Neuroscience. 2008; 9 (8):638–646. doi: 10.1038/nrn2453. [ PubMed ] [ CrossRef ] [ Google Scholar ]
- Hooley JM. Social factors in schizophrenia. Current Directions in Psychological Science. 2010; 19 (4):238–242. https://doi.org/10.1177/0963721410377597 [ Google Scholar ]
- Horn SR, Charney DS, Feder A. Understanding resilience: New approaches for preventing and treating PTSD. Experimental Neurology. 2016; 284 (Part B):119–132. https://doi.org/10.1016/j.expneurol.2016.07.002 [ PubMed ] [ Google Scholar ]
- Huettel SA, Song AW, McCarthy G. Functional magnetic resonance imaging. 2nd. Sunderland, MA: Sinauer Associates; 2008. [ Google Scholar ]
- Hunter RG, Gray JD, McEwen BS. The neuroscience of resilience. Journal of the Society for Social Work and Research (in press) [ Google Scholar ]
- Insel T. Experimental medicine [blog post] 2012 Jun 12; Retrieved from the National Institute of Mental Health website: https://www.nimh.nih.gov/about/directors/thomas-insel/blog/2012/experimental-medicine.shtml .
- Insel T, Cuthbert B, Garvey M, Heinssen R, Pine DS, Quinn K, Wang P. Research Domain Criteria (RDoC): Toward a new classification framework for research on mental disorders. American Journal of Psychiatry. 2010; 167 (7):748–751. doi: 10.1176/appi.ajp.2010.09091379. [ PubMed ] [ CrossRef ] [ Google Scholar ]
- Insel TR. The NIMH experimental medicine initiative. World Psychiatry. 2015; 14 (2):151–153. doi: 10.1002/wps.20227. [ PMC free article ] [ PubMed ] [ CrossRef ] [ Google Scholar ]
- Insel TR, Gogtay N. National Institute of Mental Health clinical trials: New opportunities, new expectations. JAMA Psychiatry. 2014; 71 (7):745–746. doi: 10.1001/jamapsychiatry.2014.426. [ PubMed ] [ CrossRef ] [ Google Scholar ]
- Insel TR, Landis SC. Twenty-five years of progress: The view from NIMH and NINDS. Neuron. 2013; 80 (3):561–567. https://doi.org/10.1016/j.neuron.2013.09.041 [ PMC free article ] [ PubMed ] [ Google Scholar ]
- Jones EG, Mendell LM. Assessing the decade of the brain. Science. 1999; 284 (5415):739–739. doi: 10.1126/science.284.5415.739. [ PubMed ] [ CrossRef ] [ Google Scholar ]
- Jones T, Price P. Development and experimental medicine applications of PET in oncology: A historical perspective. The Lancet Oncology. 2012; 13 (3):e116–e125. http://dx.doi.org/10.1016/S1470-2045(11)70183-8 [ PubMed ] [ Google Scholar ]
- Judd LL, Schettler PJ, Solomon DA, Maser JD, Coryell W, Endicott J, Akiskal HS. Psychosocial disability and work role function compared across the long-term course of Bipolar I, Bipolar II and unipolar major depressive disorders. Journal of Affective Disorders. 2008; 108 (1):49–58. http://dx.doi.org/10.1016/j.jad.2007.06.014 [ PubMed ] [ Google Scholar ]
- Kambeitz J, Cabral C, Sacchet MD, Gotlib IH, Zahn R, Serpa MH, Koutsouleris N. Detecting neuroimaging biomarkers for depression: A meta-analysis of multivariate pattern recognition studies. Biological Psychiatry. 2016; 82 (5):330–338. http://dx.doi.org/10.1016/j.biopsych.2016.10.028 [ PubMed ] [ Google Scholar ]
- Kambeitz J, Kambeitz-Ilankovic L, Leucht S, Wood S, Davatzikos C, Malchow B, Koutsouleris N. Detecting neuroimaging biomarkers for schizophrenia: A meta-analysis of multivariate pattern recognition studies. Neuropsychopharmacology. 2015; 40 (7):1742–1751. doi: 10.1038/npp.2015.22. [ PMC free article ] [ PubMed ] [ CrossRef ] [ Google Scholar ]
- Katzman R, Terry R, DeTeresa R, Brown T, Davies P, Fuld P, Peck A. Clinical, pathological, and neurochemical changes in dementia: A subgroup with preserved mental status and numerous neocortical plaques. Annals of Neurology. 1988; 23 (2):138–144. doi: 10.1002/ana.410230206. [ PubMed ] [ CrossRef ] [ Google Scholar ]
- Keshavan MS, Anderson S, Pettergrew JW. Is schizophrenia due to excessive synaptic pruning in the prefrontal cortex? The Feinberg hypothesis revisited. Journal of Psychiatric Research. 1994; 28 (3):239–265. https://doi.org/10.1016/0022-3956(94)90009-4 [ PubMed ] [ Google Scholar ]
- Keshavan MS, Eack SM, Wojtalik JA, Prasad KM, Francis AN, Bhojraj TS, Hogarty SS. A broad cortical reserve accelerates response to cognitive enhancement therapy in early course schizophrenia. Schizophrenia Research. 2011; 130 (1):123–129. https://doi.org/10.1016/j.schres.2011.05.001 [ PMC free article ] [ PubMed ] [ Google Scholar ]
- Keshavan MS, Hogarty GE. Brain maturational processes and delayed onset in schizophrenia. Development and Psychopathology. 1999; 11 (03):525–543. [ PubMed ] [ Google Scholar ]
- Keshavan MS, Mehta UM, Padmanabhan JL, Shah JL. Dysplasticity, metaplasticity, and schizophrenia: Implications for risk, illness, and novel interventions. Development and Psychopathology. 2015; 27 (2):615–635. doi: 10.1017/S095457941500019X. [ PMC free article ] [ PubMed ] [ CrossRef ] [ Google Scholar ]
- Keshavan MS, Vinogradov S, Rumsey J, Sherrill J, Wagner A. Cognitive training in mental disorders: Update and future directions. American Journal of Psychiatry. 2014; 171 (5):510–522. https://doi.org/10.1176/appi.ajp.2013.13081075 [ PMC free article ] [ PubMed ] [ Google Scholar ]
- Kimura H, Kanahara N, Takase M, Yoshida T, Watanabe H, Iyo M. A randomized, sham-controlled study of high frequency rTMS for auditory hallucination in schizophrenia. Psychiatry Research. 2016; 241 :190–194. http://dx.doi.org/10.1016/j.psychres.2016.04.119 [ PubMed ] [ Google Scholar ]
- Koutsouleris N, Borgwardt S, Meisenzahl EM, Bottlender R, Möller HJ, Riecher-Rössler A. Disease prediction in the at-risk mental state for psychosis using neuroanatomical biomarkers: Results from the FePsy Study. Schizophrenia Bulletin. 2012; 38 (6):1234–1246. doi: 10.1093/schbul/sbr145. [ PMC free article ] [ PubMed ] [ CrossRef ] [ Google Scholar ]
- Krishnan A, Williams LJ, McIntosh AR, Abdi H. Partial least squares (PLS) methods for neuroimaging: A tutorial and review. NeuroImage. 2011; 56 (2):455–475. https://doi.org/10.1016/j.neuroimage.2010.07.034 [ PubMed ] [ Google Scholar ]
- Kross E, Berman MG, Mischel W, Smith EE, Wager TD. Social rejection shares somatosensory representations with physical pain. Proceedings of the National Academy of Sciences. 2011; 108 (15):6270–6275. https://doi.org/10.1073/pnas.1102693108 [ PMC free article ] [ PubMed ] [ Google Scholar ]
- Kuo JR, Kaloupek DG, Woodward SH. Amygdala volume in combat-exposed veterans with and without posttraumatic stress disorder: A cross-sectional study. Archives of General Psychiatry. 2012; 69 (10):1080–1086. doi: 10.1001/archgenpsychiatry.2012.73. [ PubMed ] [ CrossRef ] [ Google Scholar ]
- LaBar KS, Crupain MJ, Voyvodic JT, McCarthy G. Dynamic perception of facial affect and identity in the human brain. Cerebral Cortex. 2003; 13 (10):1023–1033. doi: 10.1093/cercor/13.10.1023. [ PubMed ] [ CrossRef ] [ Google Scholar ]
- Lakhan SE, Vieira K, Hamlat E. Biomarkers in psychiatry: Drawbacks and potential for misuse. International Archives of Medicine. 2010; 3 (1):1–6. doi: 10.1186/1755-7682-3-1. [ PMC free article ] [ PubMed ] [ CrossRef ] [ Google Scholar ]
- Lewandowski KE, Ongur D, Keshavan MS. Development of novel behavioral interventions in an experimental therapeutics world: Challenges, and directions for the future. Schizophrenia Research. 2018; 192 :6–8. doi: 10.1016/j.schres.2017.06.010. [ PMC free article ] [ PubMed ] [ CrossRef ] [ Google Scholar ]
- Lozier LM, Vanmeter JW, Marsh AA. Impairments in facial affect recognition associated with autism spectrum disorders: A meta-analysis. Development and Psychopathology. 2014; 26 (4pt1):933–945. doi: 10.1017/S0954579414000479. [ PubMed ] [ CrossRef ] [ Google Scholar ]
- Lu L, Bao G, Chen H, Xia P, Fan X, Zhang J, Ma L. Modification of hippocampal neurogenesis and neuroplasticity by social environments. Experimental Neurology. 2003; 183 (2):600–609. https://doi.org/10.1016/S0014-4886(03)00248-6 [ PubMed ] [ Google Scholar ]
- Maguire EA, Gadian DG, Johnsrude IS, Good CD, Ashburner J, Frackowiak RSJ, Frith CD. Navigation-related structural change in the hippocampi of taxi drivers. Proceedings of the National Academy of Sciences of the United States of America. 2000; 97 (8):4398–4403. https://doi.org/10.1073/pnas.070039597 [ PMC free article ] [ PubMed ] [ Google Scholar ]
- Markowitz JC. There’s such a thing as too much neuroscience. The New York Times. 2016 Oct 14; Retrieved from https://www.nytimes.com/2016/10/15/opinion/theres-such-a-thing-as-too-much-neuroscience.html .
- Matto HC, Hadjiyane MC, Kost M, Marshall J, Wiley J, Strolin-Goltzman J, VanMeter JW. Functional magnetic resonance imaging clinical trial of a dual-processing treatment protocol for substance-dependent adults. Research on Social Work Practice. 2013; 24 (6):659–669. doi: 10.1177/1049731513515056. [ CrossRef ] [ Google Scholar ]
- Matto HC, Strolin-Goltzman J. Integrating social neuroscience and social work: Innovations for advancing practice-based research. Social Work. 2010; 55 (2):147–156. https://doi.org/10.1093/sw/55.2.147 [ PubMed ] [ Google Scholar ]
- Matto HC, Strolin-Goltzman J, Ballan MS. Neuroscience for social work: Current research and practice. New York, NY: Springer Publishing Company; 2014. [ Google Scholar ]
- McClure SM, Laibson DI, Loewenstein G, Cohen JD. Separate neural systems value immediate and delayed monetary rewards. Science. 2004; 306 (5695):503–507. doi: 10.1126/science.1100907. [ PubMed ] [ CrossRef ] [ Google Scholar ]
- McIntyre RS, O’Donovan C. The human cost of not achieving full remission in depression. Canadian Journal of Psychiatry. 2004; 49 (1):10–16. [ PubMed ] [ Google Scholar ]
- McLaughlin KA, Busso DS, Duys A, Green JG, Alves S, Way M, Sheridan MA. Amygdala response to negative stimuli predicts PTSD symptom onset following a terrorist attack. Depression and Anxiety. 2014; 31 (10):834–842. doi: 10.1002/da.22284. [ PMC free article ] [ PubMed ] [ CrossRef ] [ Google Scholar ]
- Messina I, Sambin M, Palmieri A, Viviani R. Neural correlates of psychotherapy in anxiety and depression: A meta-analysis. PLoS ONE. 2013; 8 (9):e74657. doi: 10.1371/journal.pone.0074657. [ PMC free article ] [ PubMed ] [ CrossRef ] [ Google Scholar ]
- Morey RA, Haswell CC, Hooper SR, De Bellis MD. Amygdala, hippocampus, and ventral medial prefrontal cortex volumes differ in maltreated youth with and without chronic posttraumatic stress disorder. Neuropsychopharmacology. 2016; 41 (3):791–801. doi: 10.1038/npp.2015.205. [ PMC free article ] [ PubMed ] [ CrossRef ] [ Google Scholar ]
- Morgane PJ, Galler JR, Mokler DJ. A review of systems and networks of the limbic forebrain/limbic midbrain. Progress in Neurobiology. 2005; 75 (2):143–160. https://doi.org/10.1016/j.pneurobio.2005.01.001 [ PubMed ] [ Google Scholar ]
- Morimoto SS, Wexler BE, Alexopoulos GS. Neuroplasticity-based computerized cognitive remediation for geriatric depression. International Journal of Geriatric Psychiatry. 2012; 27 (12):1239–1247. doi: 10.1002/gps.3776. [ PMC free article ] [ PubMed ] [ CrossRef ] [ Google Scholar ]
- Morimoto SS, Wexler BE, Liu J, Hu W, Seirup J, Alexopoulos GS. Neuroplasticity-based computerized cognitive remediation for treatment-resistant geriatric depression. Nature Communications. 2014; 5 :1–7. doi: 10.1038/ncomms5579. [ PMC free article ] [ PubMed ] [ CrossRef ] [ Google Scholar ]
- Morris SE, Cuthbert BN. Research Domain Criteria: Cognitive systems, neural circuits, and dimensions of behavior. Dialogues in Clinical Neuroscience. 2012; 14 (1):29–37. [ PMC free article ] [ PubMed ] [ Google Scholar ]
- Mortimer JA. Brain reserve and the clinical expression of Alzheimer’s disease. Geriatrics. 1997; 52 :S50–53. [ PubMed ] [ Google Scholar ]
- Mueser KT, Deavers F, Penn DL, Cassisi JE. Psychosocial treatments for schizophrenia. Annual Review of Clinical Psychology. 2013; 9 :465–497. https://doi.org/10.1146/annurev-clinpsy-050212-185620 [ PubMed ] [ Google Scholar ]
- Murphy TH, Corbett D. Plasticity during stroke recovery: From synapse to behaviour. Nature Reviews Neuroscience. 2009; 10 (12):861–872. doi: 10.1038/nrn2735. [ PubMed ] [ CrossRef ] [ Google Scholar ]
- National Institute of Mental Health (NIMH) Research Domain Criteria (RDoC) (n.d.) Retrieved from https://www.nimh.nih.gov/research-priorities/rdoc/index.shtml .
- National Institutes of Health. NIH-wide strategic plan fiscal years 2016–2020: Turning discovery into health. 2015 Retrieved from https://www.nih.gov/sites/default/files/about-nih/strategic-plan-fy2016-2020-508.pdf .
- Newby JM, McKinnon A, Kuyken W, Gilbody S, Dalgleish T. Systematic review and meta-analysis of transdiagnostic psychological treatments for anxiety and depressive disorders in adulthood. Clinical Psychology Review. 2015; 40 :91–110. https://doi.org/10.1016/j.cpr.2015.06.002 [ PubMed ] [ Google Scholar ]
- Noble KG, Houston SM, Kan E, Sowell ER. Neural correlates of socioeconomic status in the developing human brain. Developmental Science. 2012; 15 (4):516–527. doi: 10.1111/j.1467-7687.2012.01147.x. [ PMC free article ] [ PubMed ] [ CrossRef ] [ Google Scholar ]
- Novak T, Horacek J, Mohr P, Kopecek M, Skrdlantova L, Klirova M, Hoschl C. The double-blind sham-controlled study of high-frequency rTMS (20Hz) for negative symptoms in schizophrenia: Negative results. Neuroendocrinology Letters. 2006; 27 (1–2):209–213. [ PubMed ] [ Google Scholar ]
- Nusslock R, Almeida JRC, Forbes EE, Versace A, Frank E, LaBarbara EJ, Phillips ML. Waiting to win: Elevated striatal and orbitofrontal cortical activity during reward anticipation in euthymic bipolar disorder adults. Bipolar Disorders. 2012; 14 (3):249–260. doi: 10.1111/j.1399-5618.2012.01012.x.. [ PMC free article ] [ PubMed ] [ CrossRef ] [ Google Scholar ]
- Olsavsky AK, Brotman MA, Rutenberg JG, Muhrer EJ, Deveney CM, Fromm SJ, Leibenluft E. Amygdala hyperactivation during face emotion processing in unaffected youth at risk for bipolar disorder. Journal of the American Academy of Child & Adolescent Psychiatry. 2012; 51 (3):294–303. https://doi.org/10.1016/j.jaac.2011.12.008 [ PMC free article ] [ PubMed ] [ Google Scholar ]
- Pantelis C, Pantelis C, Yücel M, Wood SJ, McGorry PD, Velakoulis D. Early and late neurodevelopmental disturbances in schizophrenia and their functional consequences. Australian & New Zealand Journal of Psychiatry. 2003; 37 (4):399–406. doi: 10.1046/j.1440-1614.2003.01193.x. [ PubMed ] [ CrossRef ] [ Google Scholar ]
- Paus T, Keshavan MS, Giedd JN. Why do many psychiatric disorders emerge during adolescence? Nature Reviews Neuroscience. 2008; 9 (12):947–957. doi: 10.1038/nrn2513. [ PMC free article ] [ PubMed ] [ CrossRef ] [ Google Scholar ]
- Penadés R, González-Rodríguez A, Catalán R, Segura B, Bernardo M, Junqué C. Neuroimaging studies of cognitive remediation in schizophrenia: A systematic and critical review. World Journal of Psychiatry. 2017; 7 (1):34–43. doi: 10.5498/wjp.v7.i1.34. [ PMC free article ] [ PubMed ] [ CrossRef ] [ Google Scholar ]
- Penadés R, Pujol N, Catalán R, Massana G, Rametti G, García-Rizo C, Junqué C. Brain effects of cognitive remediation therapy in schizophrenia: A structural and functional neuroimaging study. Biological Psychiatry. 2013; 73 (10):1015–1023. https://doi.org/10.1016/j.biopsych.2013.01.017 [ PubMed ] [ Google Scholar ]
- Petrican R, Rosenbaum RS, Grady C. Neural activity patterns evoked by a spouse’s incongruent emotional reactions when recalling marriage-relevant experiences. Human Brain Mapping. 2015; 36 (10):4164–4183. doi: 10.1002/hbm.22909. [ PMC free article ] [ PubMed ] [ CrossRef ] [ Google Scholar ]
- Pittenger C, Duman RS. Stress, depression, and neuroplasticity: A convergence of mechanisms. Neuropsychopharmacology. 2008; 33 (1):88–109. doi: 10.1038/sj.npp.1301574. [ PubMed ] [ CrossRef ] [ Google Scholar ]
- Plitt M, Barnes KA, Wallace GL, Kenworthy L, Martin A. Resting-state functional connectivity predicts longitudinal change in autistic traits and adaptive functioning in autism. Proceedings of the National Academy of Sciences. 2015; 112 (48):E6699–E6706. https://doi.org/10.1073/pnas.1510098112 [ PMC free article ] [ PubMed ] [ Google Scholar ]
- Purves D, White LE, Riddle DR. Is neural development Darwinian? Trends in Neurosciences. 1996; 19 (11):460–464. https://doi.org/10.1016/S0166-2236(96)20038-4 [ PubMed ] [ Google Scholar ]
- Ramsay IS, MacDonald AW. Brain correlates of cognitive remediation in schizophrenia: Activation likelihood analysis shows preliminary evidence of neural target engagement. Schizophrenia Bulletin. 2015; 41 (6):1276–1284. https://doi.org/10.1093/schbul/sbv025 [ PMC free article ] [ PubMed ] [ Google Scholar ]
- Rao U, Chen LA, Bidesi AS, Shad MU, Thomas MA, Hammen CL. Hippocampal changes associated with early-life adversity and vulnerability to depression. Biological Psychiatry. 2010; 67 (4):357–364. https://doi.org/10.1016/j.biopsych.2009.10.017 [ PMC free article ] [ PubMed ] [ Google Scholar ]
- Rapoport JL, Giedd JN, Gogtay N. Neurodevelopmental model of schizophrenia: Update 2012. Molecular Psychiatry. 2012; 17 (12):1228–1238. doi: 10.1038/mp.2012.23. [ PMC free article ] [ PubMed ] [ CrossRef ] [ Google Scholar ]
- Rapp C. The strengths model: Case management with people suffering from severe and persistent mental illness. New York, NY: Oxford University Press; 1998. [ Google Scholar ]
- Ritchey M, Dolcos F, Eddington KM, Strauman TJ, Cabeza R. Neural correlates of emotional processing in depression: Changes with cognitive behavioral therapy and predictors of treatment response. Journal of Psychiatric Research. 2011; 45 (5):577–587. doi: 10.1016/j.jpsychires.2010.09.007. [ PMC free article ] [ PubMed ] [ CrossRef ] [ Google Scholar ]
- Rizzolatti G, Fogassi L, Gallese V. Neurophysiological mechanisms underlying the understanding and imitation of action. Nature Reviews Neuroscience. 2001; 2 (9):661–670. doi: 10.1038/35090060. [ PubMed ] [ CrossRef ] [ Google Scholar ]
- Robertson CL, Ishibashi K, Chudzynski J, Mooney LJ, Rawson RA, Dolezal BA, London ED. Effect of exercise training on striatal dopamine D2/D3 receptors in methamphetamine users during behavioral treatment. Neuropsychopharmacology. 2016; 41 (6):1629–1636. doi: 10.1038/npp.2015.331. [ PMC free article ] [ PubMed ] [ CrossRef ] [ Google Scholar ]
- Rosenzweig MR, Breedlove SM, Watson NV. Biological psychology: An introduction to behavioral and cognitive neuroscience. 4th. Sunderland, MA: Sinauer Associates; 2005. [ Google Scholar ]
- Rubin M, Shvil E, Papini S, Chhetry BT, Helpman L, Markowitz JC, Neria Y. Greater hippocampal volume is associated with PTSD treatment response. Psychiatry Research: Neuroimaging. 2016; 252 :36–39. doi: 10.1016/j.pscychresns.2016.05.001. [ PMC free article ] [ PubMed ] [ CrossRef ] [ Google Scholar ]
- Satel SL, Lilienfeld SO. Brainwashed: The seductive appeal of mindless neuroscience. New York, NY: Basic Books; 2013. [ Google Scholar ]
- Satz P. Brain reserve capacity on symptom onset after brain injury: A formulation and review of evidence for threshold theory. Neuropsychology. 1993; 7 (3):273–295. http://dx.doi.org/10.1037/0894-4105.7.3.273 [ Google Scholar ]
- Schultz RT. Developmental deficits in social perception in autism: The role of the amygdala and fusiform face area. International Journal of Developmental Neuroscience. 2005; 23 (2):125–141. https://doi.org/10.1016/j.ijdevneu.2004.12.012 [ PubMed ] [ Google Scholar ]
- Schwartz JM, Begley S. The mind and the brain: Neuroplasticity and the power of mental force. New York, NY: HarperCollins Publishers; 2003. [ Google Scholar ]
- Shapiro JR, Applegate JS. Cognitive neuroscience, neurobiology and affect regulation: Implications for clinical social work. Clinical Social Work Journal. 2000; 28 (1):9–21. https://doi.org/10.1023/A:1005139123963 [ Google Scholar ]
- Shi C, Yu X, Cheung EF, Shum DH, Chan RC. Revisiting the therapeutic effect of rTMS on negative symptoms in schizophrenia: A meta-analysis. Psychiatry Research. 2014; 215 (3):505–513. doi: 10.1016/j.psychres.2013.12.019. [ PMC free article ] [ PubMed ] [ CrossRef ] [ Google Scholar ]
- Shou H, Yang Z, Satterthwaite TD, Cook PA, Bruce SE, Shinohara RT, Sheline YI. Cognitive behavioral therapy increases amygdala connectivity with the cognitive control network in both MDD and PTSD. NeuroImage: Clinical. 2017; 14 :464–470. https://doi.org/10.1016/j.nicl.2017.01.030 [ PMC free article ] [ PubMed ] [ Google Scholar ]
- Singh I, Rose N. Biomarkers in psychiatry. Nature. 2009; 460 (7252):202–207. doi: 10.1038/460202a. [ PubMed ] [ CrossRef ] [ Google Scholar ]
- Slotema CW, Dirk Blom J, Hoek HW, Sommer IE. Should we expand the toolbox of psychiatric treatment methods to include repetitive transcranial magnetic stimulation (rTMS)? A meta-analysis of the efficacy of rTMS in psychiatric disorders. Journal of Clinical Psychiatry. 2010; 71 (7):873–884. doi: 10.4088/JCP.08m04872gre. [ PubMed ] [ CrossRef ] [ Google Scholar ]
- Small S, Hlustik P, Noll D, Genovese C, Solodkin A. Cerebellar hemispheric activation ipsilateral to the paretic hand correlates with functional recovery after stroke. Brain. 2002; 125 (7):1544–1557. https://doi.org/10.1093/brain/awf148 [ PubMed ] [ Google Scholar ]
- Smieskova R, Fusar-Poli P, Allen P, Bendfeldt K, Stieglitz R, Drewe J, Borgwardt S. Neuroimaging predictors of transition to psychosis—A systematic review and meta-analysis. Neuroscience & Biobehavioral Reviews. 2010; 34 (8):1207–1222. https://doi.org/10.1016/j.neubiorev.2010.01.016 [ PubMed ] [ Google Scholar ]
- Smith MJ, Schroeder MP, Abram SV, Goldman MB, Parrish TB, Wang X, Breiter HC. Alterations in brain activation during cognitive empathy are related to social functioning in schizophrenia. Schizophrenia Bulletin. 2015; 41 (1):211–222. doi: 10.1093/schbul/sbu023. [ PMC free article ] [ PubMed ] [ CrossRef ] [ Google Scholar ]
- Soya H, Nakamura T, Deocaris CC, Kimpara A, Iimura M, Fujikawa T, Nishijima T. BDNF induction with mild exercise in the rat hippocampus. Biochemical and Biophysical Research Communications. 2007; 358 (4):961–967. https://doi.org/10.1016/j.bbrc.2007.04.173 [ PubMed ] [ Google Scholar ]
- Sporns O, Tononi G, Kötter R. The human connectome: A structural description of the human brain. PLOS Computational Biology. 2005; 1 (4):0254–0251. doi: 10.1371/journal.pcbi.0010042. [ PMC free article ] [ PubMed ] [ CrossRef ] [ Google Scholar ]
- Squire LR, Zola-Morgan S. The medial temporal lobe memory system. Science. 1991; 253 (5026):1380–1386. doi: 10.1126/science.1896849. [ PubMed ] [ CrossRef ] [ Google Scholar ]
- Stein LI, Test MA. Alternative to mental hospital treatment: I. Conceptual model, treatment program, and clinical evaluation. Archives of General Psychiatry. 1980; 37 (4):392–397. doi: 10.1001/archpsyc.1980.01780170034003. [ PubMed ] [ CrossRef ] [ Google Scholar ]
- Stern Y. What is cognitive reserve? Theory and research application of the reserve concept. Journal of the International Neuropsychological Society. 2002; 8 :448–460. https://doi.org/10.1017/S1355617702813248 [ PubMed ] [ Google Scholar ]
- Subramaniam K, Hooker CI, Biagianti B, Fisher M, Nagarajan S, Vinogradov S. Neural signal during immediate reward anticipation in schizophrenia: Relationship to real-world motivation and function. NeuroImage: Clinical. 2015; 9 :153–163. https://doi.org/10.1016/j.nicl.2015.08.001 [ PMC free article ] [ PubMed ] [ Google Scholar ]
- Swartz JR, Knodt AR, Radtke SR, Hariri AR. A neural biomarker of psychological vulnerability to future life stress. Neuron. 2015; 85 (3):505–511. doi: 10.1016/j.neuron.2014.12.055. [ PMC free article ] [ PubMed ] [ CrossRef ] [ Google Scholar ]
- Taub E. Somatosensory deafferentation research with monkeys: Implications for rehabilitation medicine. In: Ince LP, editor. Behavioral psychology in rehabilitation medicine: Clinical applications. New York, NY: Williams & Wilkins; 1980. [ Google Scholar ]
- Taub E, Miller NE, Novack TA, Cook EW, III, Fleming WC, Nepomuceno CS, Crago JE. Technique to improve chronic motor deficit after stroke. Archives of Physical Medicine and Rehabilitation. 1993; 74 (4):347–354. [ PubMed ] [ Google Scholar ]
- Taub E, Uswatte G, Mark VW. The functional significance of cortical reorganization and the parallel development of CI therapy. Frontiers in Human Neuroscience. 2014; 8 (396):1–20. doi: 10.3389/fnhum.2014.00396. [ PMC free article ] [ PubMed ] [ CrossRef ] [ Google Scholar ]
- Thermenos HW, Juelich RJ, DiChiara SR, Mesholam-Gately RI, Woodberry KA, Wojcik J, Woo TUW. Hyperactivity of caudate, parahippocampal, and prefrontal regions during working memory in never-medicated persons at clinical high-risk for psychosis. Schizophrenia Research. 2016; 173 (1–2):1–12. https://doi.org/10.1016/j.schres.2016.02.023 [ PMC free article ] [ PubMed ] [ Google Scholar ]
- Thomaes K, Dorrepaal E, Draijer N, De Ruiter M, Elzinga B, Van Balkom A, Veltman D. Treatment effects on insular and anterior cingulate cortex activation during classic and emotional Stroop interference in child abuse-related complex post-traumatic stress disorder. Psychological Medicine. 2012; 42 (11):2337–2349. https://doi.org/10.1017/S0033291712000499 [ PubMed ] [ Google Scholar ]
- Tottenham N, Galván A. Stress and the adolescent brain. Neuroscience & Biobehavioral Reviews. 2016; 70 :217–227. http://dx.doi.org/10.1016/j.neubiorev.2016.07.030 [ PMC free article ] [ PubMed ] [ Google Scholar ]
- Uljarevic M, Hamilton A. Recognition of emotions in autism: A formal meta-analysis. Journal of Autism and Developmental Disorders. 2013; 43 (7):1517–1526. doi: 10.1007/s10803-012-1695-5. [ PubMed ] [ CrossRef ] [ Google Scholar ]
- U.S. Burden of Disease Collaborators. The state of U.S. health, 1990–2010: Burden of diseases, injuries, and risk factors. JAMA. 2013; 310 (6):591–606. doi: 10.1001/jama.2013.13805. [ PMC free article ] [ PubMed ] [ CrossRef ] [ Google Scholar ]
- Van Den Heuvel MP, Pol HEH. Exploring the brain network: A review on resting-state fMRI functional connectivity. European Neuropsychopharmacology. 2010; 20 (8):519–534. https://doi.org/10.1016/j.euroneuro.2010.03.008 [ PubMed ] [ Google Scholar ]
- van der Werff S, van den Berg S, Pannekoek J, Elzinga B, Van Der Wee N. Neuroimaging resilience to stress: A review. Frontiers in Behavioral Neuroscience. 2013; 7 (39) doi: 10.3389/fnbeh.2013.00039. [ PMC free article ] [ PubMed ] [ CrossRef ] [ Google Scholar ]
- Van Praag H, Shubert T, Zhao C, Gage FH. Exercise enhances learning and hip-pocampal neurogenesis in aged mice. Journal of Neuroscience. 2005; 25 (38):8680–8685. https://doi.org/10.1523/JNEUROSCI.1731-05.2005 [ PMC free article ] [ PubMed ] [ Google Scholar ]
- Wackerhagen C, Wüstenberg T, Mohnke S, Erk S, Veer IM, Kruschwitz JD, Schweiger JI. Influence of familial risk for depression on cortico-limbic connectivity during implicit emotional processing. Neuropsychopharmacology. 2017; 42 :1729–1738. doi: 10.1038/npp.2017.59. [ PMC free article ] [ PubMed ] [ CrossRef ] [ Google Scholar ]
- Walter A, Suenderhauf C, Harrisberger F, Lenz C, Smieskova R, Chung Y, Bend-feldt K. Hippocampal volume in subjects at clinical high-risk for psychosis: A systematic review and meta-analysis. Neuroscience & Biobehavioral Reviews. 2016; 71 :680–690. https://doi.org/10.1016/j.neubiorev.2016.10.007 [ PubMed ] [ Google Scholar ]
- Wang X, Cao Q, Wang J, Wu Z, Wang P, Sun L, Wang Y. The effects of cognitive-behavioral therapy on intrinsic functional brain networks in adults with attention-deficit/hyperactivity disorder. Behaviour Research and Therapy. 2016; 76 :32–39. https://doi.org/10.1016/j.brat.2015.11.003 [ PubMed ] [ Google Scholar ]
- Whitton AE, Treadway MT, Pizzagalli DA. Reward processing dysfunction in major depression, bipolar disorder and schizophrenia. Current Opinion in Psychiatry. 2015; 28 (1):7–12. doi: 10.1097/YCO.0000000000000122. [ PMC free article ] [ PubMed ] [ CrossRef ] [ Google Scholar ]
- Wojtalik JA, Smith MJ, Keshavan MS, Eack SM. A systematic and meta-analytic review of neural correlates of functional outcome in schizophrenia. Schizophrenia Bulletin. 2017; 43 (6):1329–1347. doi: 10.1093/schbul/sbx008. [ PMC free article ] [ PubMed ] [ CrossRef ] [ Google Scholar ]
- Wolf RC, Höse A, Frasch K, Walter H, Vasic N. Volumetric abnormalities associated with cognitive deficits in patients with schizophrenia. European Psychiatry. 2008; 23 (8):541–548. https://doi.org/10.1016/j.eurpsy.2008.02.002 [ PubMed ] [ Google Scholar ]
- Woo CW, Chang LJ, Lindquist MA, Wager TD. Building better biomarkers: Brain models in translational neuroimaging. Nature Neuroscience. 2017; 20 (3):365–377. doi: 10.1038/nn.4478. [ PMC free article ] [ PubMed ] [ CrossRef ] [ Google Scholar ]
- World Health Organization. Investing in mental health: Evidence for action. 2013 Retrieved from http://apps.who.int/iris/bitstream/10665/87232/1/9789241564618_eng.pdf?uap1 .
- Yamada M, Hirao K, Namiki C, Hanakawa T, Fukuyama H, Hayashi T, Murai T. Social cognition and frontal lobe pathology in schizophrenia: A voxel-based morphometric study. Neuroimage. 2007; 35 (1):292–298. https://doi.org/10.1016/j.neuroimage.2006.10.046 [ PubMed ] [ Google Scholar ]
- Zhong M, Wang X, Xiao J, Yi J, Zhu X, Liao J, Yao S. Amygdala hyper-activation and prefrontal hypoactivation in subjects with cognitive vulnerability to depression. Biological Psychology. 2011; 88 (2):233–242. https://doi.org/10.1016/j.biopsycho.2011.08.007 [ PubMed ] [ Google Scholar ]
- Share full article
Advertisement
Supported by
Study Suggests Genetics as a Cause, Not Just a Risk, for Some Alzheimer’s
People with two copies of the gene variant APOE4 are almost certain to get Alzheimer’s, say researchers, who proposed a framework under which such patients could be diagnosed years before symptoms.

By Pam Belluck
Scientists are proposing a new way of understanding the genetics of Alzheimer’s that would mean that up to a fifth of patients would be considered to have a genetically caused form of the disease.
Currently, the vast majority of Alzheimer’s cases do not have a clearly identified cause. The new designation, proposed in a study published Monday, could broaden the scope of efforts to develop treatments, including gene therapy, and affect the design of clinical trials.
It could also mean that hundreds of thousands of people in the United States alone could, if they chose, receive a diagnosis of Alzheimer’s before developing any symptoms of cognitive decline, although there currently are no treatments for people at that stage.
The new classification would make this type of Alzheimer’s one of the most common genetic disorders in the world, medical experts said.
“This reconceptualization that we’re proposing affects not a small minority of people,” said Dr. Juan Fortea, an author of the study and the director of the Sant Pau Memory Unit in Barcelona, Spain. “Sometimes we say that we don’t know the cause of Alzheimer’s disease,” but, he said, this would mean that about 15 to 20 percent of cases “can be tracked back to a cause, and the cause is in the genes.”
The idea involves a gene variant called APOE4. Scientists have long known that inheriting one copy of the variant increases the risk of developing Alzheimer’s, and that people with two copies, inherited from each parent, have vastly increased risk.
The new study , published in the journal Nature Medicine, analyzed data from over 500 people with two copies of APOE4, a significantly larger pool than in previous studies. The researchers found that almost all of those patients developed the biological pathology of Alzheimer’s, and the authors say that two copies of APOE4 should now be considered a cause of Alzheimer’s — not simply a risk factor.
The patients also developed Alzheimer’s pathology relatively young, the study found. By age 55, over 95 percent had biological markers associated with the disease. By 65, almost all had abnormal levels of a protein called amyloid that forms plaques in the brain, a hallmark of Alzheimer’s. And many started developing symptoms of cognitive decline at age 65, younger than most people without the APOE4 variant.
“The critical thing is that these individuals are often symptomatic 10 years earlier than other forms of Alzheimer’s disease,” said Dr. Reisa Sperling, a neurologist at Mass General Brigham in Boston and an author of the study.
She added, “By the time they are picked up and clinically diagnosed, because they’re often younger, they have more pathology.”
People with two copies, known as APOE4 homozygotes, make up 2 to 3 percent of the general population, but are an estimated 15 to 20 percent of people with Alzheimer’s dementia, experts said. People with one copy make up about 15 to 25 percent of the general population, and about 50 percent of Alzheimer’s dementia patients.
The most common variant is called APOE3, which seems to have a neutral effect on Alzheimer’s risk. About 75 percent of the general population has one copy of APOE3, and more than half of the general population has two copies.
Alzheimer’s experts not involved in the study said classifying the two-copy condition as genetically determined Alzheimer’s could have significant implications, including encouraging drug development beyond the field’s recent major focus on treatments that target and reduce amyloid.
Dr. Samuel Gandy, an Alzheimer’s researcher at Mount Sinai in New York, who was not involved in the study, said that patients with two copies of APOE4 faced much higher safety risks from anti-amyloid drugs.
When the Food and Drug Administration approved the anti-amyloid drug Leqembi last year, it required a black-box warning on the label saying that the medication can cause “serious and life-threatening events” such as swelling and bleeding in the brain, especially for people with two copies of APOE4. Some treatment centers decided not to offer Leqembi, an intravenous infusion, to such patients.
Dr. Gandy and other experts said that classifying these patients as having a distinct genetic form of Alzheimer’s would galvanize interest in developing drugs that are safe and effective for them and add urgency to current efforts to prevent cognitive decline in people who do not yet have symptoms.
“Rather than say we have nothing for you, let’s look for a trial,” Dr. Gandy said, adding that such patients should be included in trials at younger ages, given how early their pathology starts.
Besides trying to develop drugs, some researchers are exploring gene editing to transform APOE4 into a variant called APOE2, which appears to protect against Alzheimer’s. Another gene-therapy approach being studied involves injecting APOE2 into patients’ brains.
The new study had some limitations, including a lack of diversity that might make the findings less generalizable. Most patients in the study had European ancestry. While two copies of APOE4 also greatly increase Alzheimer’s risk in other ethnicities, the risk levels differ, said Dr. Michael Greicius, a neurologist at Stanford University School of Medicine who was not involved in the research.
“One important argument against their interpretation is that the risk of Alzheimer’s disease in APOE4 homozygotes varies substantially across different genetic ancestries,” said Dr. Greicius, who cowrote a study that found that white people with two copies of APOE4 had 13 times the risk of white people with two copies of APOE3, while Black people with two copies of APOE4 had 6.5 times the risk of Black people with two copies of APOE3.
“This has critical implications when counseling patients about their ancestry-informed genetic risk for Alzheimer’s disease,” he said, “and it also speaks to some yet-to-be-discovered genetics and biology that presumably drive this massive difference in risk.”
Under the current genetic understanding of Alzheimer’s, less than 2 percent of cases are considered genetically caused. Some of those patients inherited a mutation in one of three genes and can develop symptoms as early as their 30s or 40s. Others are people with Down syndrome, who have three copies of a chromosome containing a protein that often leads to what is called Down syndrome-associated Alzheimer’s disease .
Dr. Sperling said the genetic alterations in those cases are believed to fuel buildup of amyloid, while APOE4 is believed to interfere with clearing amyloid buildup.
Under the researchers’ proposal, having one copy of APOE4 would continue to be considered a risk factor, not enough to cause Alzheimer’s, Dr. Fortea said. It is unusual for diseases to follow that genetic pattern, called “semidominance,” with two copies of a variant causing the disease, but one copy only increasing risk, experts said.
The new recommendation will prompt questions about whether people should get tested to determine if they have the APOE4 variant.
Dr. Greicius said that until there were treatments for people with two copies of APOE4 or trials of therapies to prevent them from developing dementia, “My recommendation is if you don’t have symptoms, you should definitely not figure out your APOE status.”
He added, “It will only cause grief at this point.”
Finding ways to help these patients cannot come soon enough, Dr. Sperling said, adding, “These individuals are desperate, they’ve seen it in both of their parents often and really need therapies.”
Pam Belluck is a health and science reporter, covering a range of subjects, including reproductive health, long Covid, brain science, neurological disorders, mental health and genetics. More about Pam Belluck
The Fight Against Alzheimer’s Disease
Alzheimer’s is the most common form of dementia, but much remains unknown about this daunting disease..
How is Alzheimer’s diagnosed? What causes Alzheimer’s? We answered some common questions .
A study suggests that genetics can be a cause of Alzheimer’s , not just a risk, raising the prospect of diagnosis years before symptoms appear.
Determining whether someone has Alzheimer’s usually requires an extended diagnostic process . But new criteria could lead to a diagnosis on the basis of a simple blood test .
The F.D.A. has given full approval to the Alzheimer’s drug Leqembi. Here is what to know about i t.
Alzheimer’s can make communicating difficult. We asked experts for tips on how to talk to someone with the disease .
Neuroscience
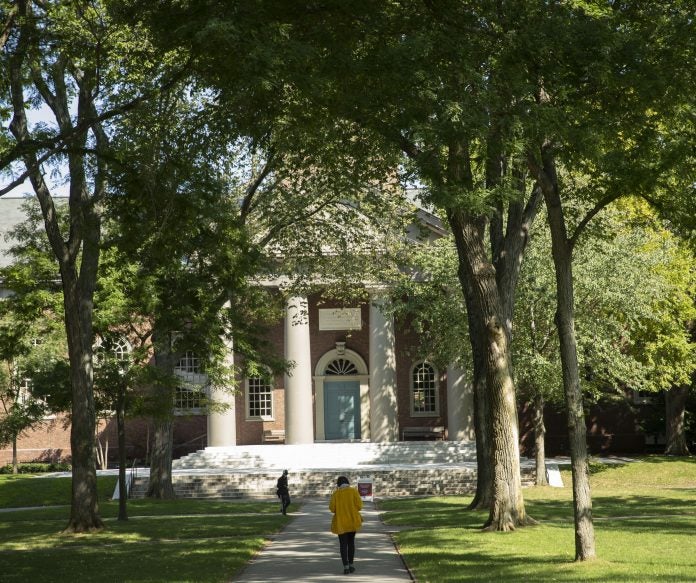
Undergraduate Program
Neuroscience, the study of the nervous system, is a field that investigates the biological mechanisms that underlie behavior and how brains process information. The study of neuroscience provides both a broad scientific training and a deep understanding of the biology of the nervous system. Given the diversity of interests in this field, the only prerequisite for students entering this concentration is an intense curiosity about the brain.
The Program in Neuroscience is an inter-departmental Ph.D. program dedicated to training Ph.D.s in neuroscience. The program provides students with the instruction, research experience, and mentoring they need to become leaders in research and education. The program offers students options for thesis research with neuroscientists in departments throughout the University, including in labs based on the Cambridge campus and at Harvard-affiliated hospitals. The enormous number and diversity of affiliated labs means that students have a wide range of options in choosing research experiences.
- Reference Manager
- Simple TEXT file
People also looked at
Hypothesis and theory article, what neuroscientists think, and don’t think, about consciousness.
- Department of Anatomy and Physiology, University of Melbourne, Parkville, MO, Australia
The approach the majority of neuroscientists take to the question of how consciousness is generated, it is probably fair to say, is to ignore it. Although there are active research programs looking at correlates of consciousness, and explorations of informational properties of what might be relevant neural ensembles, the tacitly implied mechanism of consciousness in these approaches is that it somehow just happens. This reliance on a “magical emergence” of consciousness does not address the “objectively unreasonable” proposition that elements that have no attributes or properties that can be said to relate to consciousness somehow aggregate to produce it. Neuroscience has furnished evidence that neurons are fundamental to consciousness; at the fine and gross scale, aspects of our conscious experience depend on specific patterns of neural activity – in some way, the connectivity of neurons computes the features of our experience. So how do we get from knowing that some specific configurations of cells produce consciousness to understanding why this would be the case? Behind the voltages and currents electrophysiologists measure is a staggeringly complex system of electromagnetic fields – these are the fundamental physics of neurons and glia in the brain. The brain is entirely made of electromagnetism (EM) phenomena from the level of the atoms up. The EM field literally manifests the computations, or signaling, or information processing/activities performed by connected cellular ensembles that generate a 1st-person perspective. An investigation into the EM field at the cellular scale provides the possibility of identifying the outward signs of a mechanism in fundamental terms (physics), as opposed to merely describing the correlates of our mental abstractions of it.
To the theoretical question, Can you design a machine to do whatever a brain can do? The answer is this: If you will specify in a finite and unambiguous way what you think a brain does do with information, then we can design a machine to do it. Pitts and I have proven this construction. But can you say what you think brains do? McCulloch (1965)
Neuroscientists and the Neuroscience of Consciousness
Neuroscience research, led by the funding that supports it, is dominated by research into disorders of the nervous system. The pursuit of treatments and cures (and the research efforts into understanding the normal function of the brain) builds on decades of discovery into all levels of neural organization – seemingly none of it reliant on knowing very much at all about consciousness. Studies of how consciousness is generated, and why it has the characteristics it does, is nevertheless a focus of considerable interest and effort. There is no consensus about how it is generated, or how best to approach the question, but all investigations start with the incontrovertible premise that consciousness comes about from the action of the brain.
A key driver of a general understanding of how the nervous system works are the discoveries relating to how signals are combined and transmitted by neurons. From Golgi’s and Ramon-y-Cajal’s insights that neurons are morphologically specialized to form extensive interconnectivity ( Glickstein, 2006 ), a sample of Nobel prizes provides an effective summary of the progress in our understanding of how neurons perform the functions of the nervous system: to Eccles, Hodgkin, and Huxley for “discoveries concerning the ionic mechanisms involved in excitation and inhibition in the peripheral and central portions of the nerve cell membrane”; to Katz, von Euler, and Axelrod for “discoveries concerning the humoral transmitters in the nerve terminals and the mechanism for their storage, release and inactivation,” and to Neher and Sakmann for “discoveries concerning the function of single ion channels in cells.” These discoveries were crucial for understanding how neurons generate, transmit and integrate biological signals and have had an understandably huge impact across all of neuroscience ( Eccles, 1982 ; Valenstein, 2002 ; Augustine and Kasai, 2007 ; Reyes, 2019 ). Central to this understanding is the deep insight of how the biophysics of ionic movement across the neuronal membrane expresses the action potential. The significance and influence of this discovery is captured admirably in McComas’ history “Galvani’s Spark”:
“The nerve impulse is the mechanism by which the brain conducts its affairs, the currency for all its transactions” McComas (2011)
Another immensely significant and influential discovery points directly to how the action of neuronal circuits identifies features in the visual scene that form elements of visual perception. By mapping the adequate stimuli of receptive fields in the visual system, Hubel and Wiesel [see Constantine-Paton (2008) and Wurtz (2009) for review] discovered that the connectivity between neurons along the pathway dictates that the receptive fields in visual cortex are tuned to features such as edges and boundaries. Neuronal receptive fields are a product of the inputs they receive so it seems very reasonable to consider that the neurons of the visual pathway, by virtue of their signaling configuration, compute (in some sense) features of the perceptual experience.
In its generalized recent form, what has become “computation by synaptic connectivity” is accepted as the basis of nervous system function. This has convergent support from formal computing. In recent times, digital computers implementing artificial neural networks show that the simple learning rules that define an optimization process cause (for example) convolution kernels to converge from an initial random configuration to a collection of filters that are optimally activated by various oriented edges and simple luminance distributions that comprise the features of the images ( Linsker, 1986a , b , c ; Olshausen and Field, 1996 ; Gregor and LeCun, 2010 ). Deeper layers of these networks become maximally activated by more abstract features of images. These properties, as well as the reliance of learning (network adaptation) through interaction with stimuli, and the lack of explicit specified initial connectivity (and their eventual accuracy), seem to embody many attributes of biological visual systems. This understanding of the brain has become a powerful driver of modern progress in artificial intelligence ( LeCun et al., 2015 ; O’Shea and Nash, 2015 ; Schmidhuber, 2015 ).
It is probably difficult to overstate the influence that the rapid development and exploration of formal computation (and especially digital computing) has had on our ideas about how the brain works. Rather than taking formal computation as a metaphor, it is not at all uncommon to hear present day brain scientists ask not whether the brain is any sort of computer but rather, what sort of computer is it. 1 For example, the recent enterprise of connectomics assumes not only that the computational aspects of the brain are sufficiently represented in the connectome, but also that the revealed connectome will provide the best possibility of answering questions about how the brain works. The claim that “ Neuroscience would be much easier if we had a detailed circuit diagram of the brain ” ( Martin, 2006 ) may be true, but it is not so clear that the connectome’s explanatory power would extend to how the brain generates consciousness.
What is interesting is that a scientific account of consciousness need form no explicit part of what motivated the cited progress in neuroscience. Indeed, attention to consciousness has woven a history of considerable scientific-cultural controversy into it. Neuroscience is a relative late comer to the question of how consciousness arises, and it is only in the last decades that this topic obtained a level of legitimacy within neuroscience research. This transformation can arguably be specifically dated to the 1990 work of Francis Crick (another Nobel Laureate) and Kristoff Koch that gave birth to the (neurobiological) “correlates-of-consciousness” empirical paradigm ( Crick and Koch, 1990 ) and its descendants. By organizing the science around isolation of observational “correlates-of” consciousness, a physical science could finally get permission to deal with consciousness without its related funding application being tainted by a historically “career-limiting” direct attack on what was then a taboo explanandum in the physical sciences: the 1st-person perspective (1PP) ( Wallace, 2000 ).
This transition of the science of consciousness into the physical sciences is now entering its fourth successful decade of relief from a long era of explicit-funding-pariah-hood in the physical sciences ( Koch, 2019 ; Seth, 2021 ). The centuries of prior history of attempts to explain consciousness, in ways too numerous to address here, have been swamped by the knowledge delivered by the 30 years of neuroscience’s active presence in the area. This has occurred despite it being successfully ignored by the bulk of mainstream neuroscientists. In the “correlates-of” paradigm we all set aside the fact that top-down observational correlates do not reveal principled explanations ( Seth, 2009 ). For three decades we have elected to live with that limitation while making impressive progress in exploring for the outward signs of delivery of consciousness by brain activity. Strategically, the “correlates of” paradigm has been a highly effective way to make progress. What it has not done, however, is conclusively isolate the originating principle that might predict brain material’s 1PP.
By its nature, and for good reason, the “correlates-of” paradigm bypasses the true significance of the 1990 event. To see the significance more clearly, consider that producing an abstract “3rd-person-perspective” (3PP) model, that is predictive of observable properties of nature, is the normal, familiar end of the concerns of a physical science. But in 1990 this changed. The ultimate target of the neuroscience of consciousness is an account of “what it is like to be” the studied nature. This is a categorically distinct, novel kind of explanandum. The burden of accounting for the 1PP falls on neuroscience because the human brain’s cranial central excitable cell biology somehow delivers the only instance of a 1PP known to science (this excludes the spinal cord and the peripheral nervous system as originators of subjective experience itself). The human brain’s 1PP is the reason we have a science of consciousness. This is not a “business-as-usual” scientific context. No other physical science has this confluence of circumstances and obligation. For example, no Perovskite geologist is required to account for “what it is like to be Perovskite.” Not so for the neuroscientist and tissue based on excitable cells made of atoms from the same table of elements used in Perovskite.
To deal with this unprecedented explanatory target, the “correlates-of” empirical paradigm was established as an ersatz form of explanation of the 1PP by procedurally rendering it in the familiar, centuries-old 3PP form. It does this in practice by explicitly studying a “correlate of a 1PP report .” This is the extra distancing from the target that attracts the “correlates-of” moniker. The 1PP itself is not observed by the attending scientists . Instead, the “report” is observed as a highly curated form of hearsay evidence. As a successful empirical method, it has resulted in the bourgeoning and sophisticated knowledge of consciousness that has arisen in the last three decades. However, the intrinsic indirectness and non-uniqueness of the evidence undermines, possibly fatally and indefinitely, the goal of understanding how brains produce consciousness.
If neuroscience is to make a contribution to this rather daunting foundational issue, what might be the form of a solution to the origin of a 1PP? Exactly what is it that brain tissue is “being”? The fundamental physics of electromagnetism (EM) is a very attractive candidate but, effectively, an undiscovered country in the life of the mainstream neuroscientist. Excluding explicit attention to the fundamental physics of the brain has clearly not prevented huge advances in neuroscience but may be precluding investigation of how the brain generates consciousness. In this reframing of approach, what is proposed here isn’t an EM theory of consciousness (EM ToC) but a case for why a ToC should first be sought, by neuroscience, in the EM phenomena of brains.
Electromagnetism and the Science of Consciousness
The standard model of particle physics is about twice the age of the modern “correlates-of” form of the science of consciousness ( Cottingham and Greenwood, 2007 ; Rich, 2010 ). In it, physics has already determined what our biosphere and everything in it is made of. It is effectively entirely electromagnetism (electromagnetic fields). This idea applies to anything made of atoms from the table of the elements at a spatiotemporal scale above that of the atomic particles comprising atoms (electrons and nuclei). At the atomic level and above, we and our host environment are defined by three things: space, an EM field system impressed on space (due to subatomic charge and spin content tightly bound up with the subatomic mass), and a gravitational field impressed on space (due to sub-atomic mass, functionally inert in context because it is more than 16 orders of magnitude weaker in force transmission than EM). In rough terms, at the intra-atomic scale, EM fields occupy the space occupied by an atom to the extent of at least 14,999 parts in 15,000. The remaining “1 part” is the interior of electrons and nuclei. When you add in the space between atoms, the proportion of overall spatial occupancy by EM fields is far higher. We humans are nearly entirely EM field objects. In our context of the brain, when we use the words “material” or “physical,” these words (abstractions) refer to EM phenomena.
Therefore, the question “What is it that we are ‘being’?” has an answer in the standard model: “We are ‘being’ EM fields from the atomic level up.” Brevity demands that we avoid going into a discourse on the details, defending it right down into the subatomic intricacies and across the four fundamental force quadrants of the standard model. The standard model’s EM-quadrant/atomic basis of our biosphere is just a basic, well established and proved fact of the physics. More important is how this basic fact impacts a science of consciousness. What is it like to “be” EM fields when the EM fields are configured in the form of a healthy, awake, alert human brain? To be such a configuration of EM fields is, under the right conditions, to be conscious. That is, fundamental physics has already, prima facie , determined a bottom-up (fundamental) origin of a 1PP: EM fields. There is literally nothing else there but a functionally irrelevant gravitational field and space. The endogenous EM field expressed by the atomic-level componentry of the brain entirely fills the space occupied by a brain, spilling out from its generating tissue into the surrounding tissue and beyond the skull. An EM ToC merely points out that basic fact and explicitly holds particular aspects of “the brain as an EM field” accountable for a 1PP. As a (bottom-up) claim made with well-established fundamental physics, such a proposal has a clear critical advantage, giving it priority.
What the fundamental physics lacks is an explanation of where EM’s potential for a 1PP comes from, and what specific patterning of brain EM is necessary and sufficient to create a 1PP of a specific kind (qualia or “qualitative feel”) and specific degree (spatial extension, granular resolution, duration, and intensity). Here we set aside this lack as a secondary issue. In terms of a strategic direction for the science, what matters is the obvious centrality of EM fields as the prime candidate for a route to a full explanation of consciousness in fundamental physics terms yet to be formulated. We are all familiar with the EM field system of the brain. Every measurement ever made in support of any ToC involves accessing and characterizing EM properties of the brain (more on this later).
The EM field system impressed on space by brain tissue is therefore not a side effect of cells made of something else. The entire tissue is a single, unitary EM field system impressed on space with atomic-level resolution. For example, there is no special substance that is a neuron. A neuron is a collection of EM fields “behaving neuron-ly” to an observer made of EM fields. “Chemical” or “chemical reaction,” or “chemical pathway” is a reference to EM field activity. “Mechanical” (such as sound propagation/transduction/phonons, or cell deformation) is also an EM phenomenon. “Electro-chemical” is also selecting phenomena entirely comprised of EM. “Quantum mechanics” is not a substance. It is a set of (wave-equation-based) quantizing constraints on EM field expression (such as that determining the electron orbitals in an atom). “Chemical potential” is a population statistic depicting average EM field properties for particular collections of atoms in relation to each other. “Action potentials” are a system of EM field dynamics propagating slowly through space longitudinally following neuronal cell membrane (also an EM field construct). Synapse activity (“electrical” and “chemical”) is an EM field phenomenon. The familiar electrophysiological measurements made in brain tissue detect “total field” in the brain that is a result of the vector-field superposition of myriad individual atomic/molecular field sources that superpose to dominate (spatially, temporally, and in intensity) the underlying atomic/molecular EM field “noise” found at any point in space. “Electrical current” is a transit of an EM field system through space. Ultraweak biophoton and thermal (heat) radiation is also an EM field phenomenon originating in the same system of atomic sources. Diffusion is a collection of randomly colliding atomic EM field systems bouncing off each other due to EM field-based repulsion. To “touch something” with your finger is to engage in an interaction between the EM field system of a finger surface and the EM field of the touched entity.
There is nothing left to describe in a brain that is not EM fields until we get into the interior of the subatomic constituents of atoms. This property is not limited merely to the brain. The pancreas and the heart (or any other organ) are also EM field objects from the atomic level up. What distinguishes the brain’s EM field system from that of any other organ is that its cells can generate an EM configuration conferring the 1PP for humans. Our “Perovskite” rock (above) is also an EM field object, presumably (we conjecture) lacking the specifics of EM field expression that results in a 1PP for the rock.
We can apply the same considerations to previous attempts to explain consciousness using “top-down” abstractions of aggregations of particular formations of EM fields construed as “information,” “signal processing,” “computation,” “thalamocortical loop,” “entropy dynamics,” “resonance,” “reciprocal loops,” “function,” “behavior” and many others. These are all “correlates-of” labels applied to refer to the organization and properties of EM fields. It doesn’t matter whether such depictions of brain tissue operate at molecular/atomic, subcellular, cell organelle, cellular, cell ensemble, cell population, or whole-tissue level. In every case it is EM fields that literally manifest the observable property hypothesized to originate a 1PP. Locating and describing these top-down field-abstractions as “correlates” has, for 30 years, been held up as a route to an explanation of consciousness. But such abstracted “top-down” features that correlate with aspects of consciousness seem to have no explanatory relevance to, or information concerning, the causal basis for having any form of consciousness. An EM ToC seeks an explanation in a separate fundamental physics account of how “being” (bottom-up) EM fields actually originates a 1PP.
These considerations of the state of the science extend even into the long history of EM field theories of consciousness. For interested readers the history and scope of existing EM ToC can be found through reviews ( Jones, 2013 , 2017 ; Pockett, 2013 ). But the details therein are not germane here. In reality all ToC (EM and otherwise) are actually, ultimately, EM field theories sometimes disguised out of view by a chosen kind of abstraction and then empirically supported by measurements also disguising their ultimate EM basis in tissue. We are proposing that we all collectively converge on the reality that it is actually EM fields that originate the 1PP, and engage with fundamental physics in whatever novel manner is necessary to hold it accountable for the origins of a 1PP.
Notice that no existing theory of consciousness is invalidated by this proposal. It is quite possible that one of the plethora of “correlates” is right! This is not contested here. What this article argues is that the “correlate” can be right and yet deliver no actual explanation (no principled account of the origin of the unique explanandum). This is because the EM basis of the correlate is the actual source of the origin of the claimed correlate’s connection to a 1PP.
Consciousness From Computation
We can further explore the utility of EM in providing explanation of the origin of consciousness by consideration of ToC that do not posit any role for EM. If there are no features, other than those related to signaling between its constituent cells, that neurons contribute to how the brain works, a parsimonious explanation for consciousness is that it too is the result of signal processing (a specific form of computation). This is entirely consistent with the accumulated evidence from the history of studying the brain, which has reinforced, at coarse and fine scales, that the details of conscious experience are associated with the details of brain activity. As previously noted, the evident truth of this does not provide an explanation of why it is so.
The idea that consciousness arises from processing signals (of the now well-defined and well-understood neuronal forms) would give rise to the phenomenon of a 1PP can be called “strong emergence” (or “magical emergence”) because there is, currently, no reason to hold that such a phenomenon would, should, or could follow from the known properties of the system’s constituents ( Bedau, 1997 ; Chalmers, 2006 ; O’Connor, 2020 ). This gap in the explanatory sequence has been discussed for as long as the nature of the mind has been considered ( Levine, 1983 ; Van Gulick, 2018 ), and has more recently been characterized by David Chalmers as the “hard problem”: “ Why should physical processing give rise to a rich inner life at all? It seems objectively unreasonable that it should, and yet it does ” ( Chalmers, 1995 , 1996 , 1997 ).
The computational view renders consciousness either a rather unimportant feature of brain function or a causally inert epiphenomenon inhering in it. If everything the nervous system does is computation, and thus computation does everything, then there would seem to be no need for consciousness. This disconnects the computational or symbolic representation of brain operation from the physics of the system it represents (the EM physics of nervous system signaling). In other science disciplines, digital models or simulations are used to represent the known and hypothesized attributes and relationships between the elements of a system. Sufficiently accurate and comprehensive models (of the 3PP kind discussed earlier) allow prediction that corresponds to the performance/properties of the real systems (the ones being modeled) and can validate assumptions and hypotheses used in the model.
In the brain sciences, however, the models take on a very different expectation: the simulations are doing exactly the same transformations of signals and data that they are representing in the real system, so must display all phenomena of the system being represented. For example, a computer model of combustion might indicate, numerically, how much heat is produced, but it doesn’t get hot - the simulation uses abstract representations, not the actual physics of combustion. Neuroscientists are entitled to ask what goes missing, in the sense of the heat in the combustion example, when the physics of brain signaling is thrown out and replaced by the physics of a computer. Is the computer and its model really contacting all brain phenomena? If there is something missing, how would we know? What procedure might we use to find out? This is the challenge posed by the McCulloch quote ( McCulloch, 1965 ) at the start of this article.
In brain sciences that study consciousness within the paradigm of computation, there is no perceived need to relate the model’s results to the actual physics of brains and neurons. In the current neuroscience paradigm, the physics of brain cells can be entirely ignored once we have sufficient data on activations and connectivity to accurately mimic the signal processing apparently performed by brain signaling physics. This “abstracting away” of the underlying fundamental physics implies that consciousness will emerge from the analogous (or informationally equivalent) simulation of a model of the signal processing that happens in brains. One of the difficulties with accepting this kind of strong emergence as an explanation for consciousness is that it is unclear how to proceed from this position to a deeper understanding of how this happens. “Emergent” as an explanation has the same value as using the term “happens” ( Kelly, 1994 ). Our normal expectation of explanations has been classified as “weak emergence” because they say something about why things happen in terms of a mechanistic link between the attributes and actions of the relevant parts and the phenomena they generate. This contrasts with strong emergence, which is literally defined as a form of explanatory failure ( Bedau, 1997 ; Chalmers, 2006 ; O’Connor, 2020 ). If consciousness is properly explained, then it would be transformed into weak emergence: a predictable whole resulting from the understood properties of its parts.
But whether the tendency of this “hard problem” to elicit a reliance on strong emergence is seen as a fundamental, or large, or illusory, obstacle to understanding consciousness, it has little bearing on the research being undertaken by neuroscientists working in the standard, albeit tacit, mode of scientific investigation: obtaining 3PP descriptions of nervous system structure and function. Neuroscientists may well suspect that consciousness emerges (somehow) at some higher level of organization to the level of explanation they are pursuing, so a solution is not required of them. This exonerates neuroscientists for being unconcerned about their discipline’s ignorance of consciousness, and absolves them from exploring why EM ToC might offer plausible explanations. We pose that EM should not be ignored because it is actually at the heart of all phenomena in the nervous system, and when neuroscientists measure brain phenomena, the action potentials measured as transmembrane voltage, the “local field potential” (LFP), EEG, and MEG, and so forth, are all aspects of the fine-scale EM phenomena that actually underline the brain’s signaling systems, our characterization of them, and our stimulation of them when we intervene in brain function. To ignore explicit attention to EM, by subsuming it into simplified measurements applied to an abstraction of it, is to cast an irreversible pall of strong emergence over the explanatory discourse of the science of consciousness.
In recent times it has become possible to see EM field interactions within tissue having a direct effect on neuronal excitation. This new signaling mechanism, “ephaptic transmission” shows the causal power of the brain’s endogenous EM fields on its own neural activation. For example, the EM fields associated with neural activity have been shown to generate traveling waves of neuronal excitation in hippocampal pyramidal neurons ( Chiang et al., 2019 ). This characterization of ephaptic transmission in the hippocampus is significant as it implies that EM field propagation can traverse considerable distances in laminated (spatially coherent) and synchronized (temporally coherent) neuronal assemblies - and laminated neuronal assemblies are a fundamental architectural principle across the central nervous system (CNS). This real example of EM fields having a direct effect on neural signaling reveals another advantage inherent in an EM field approach: the provision of a fundamental causal mechanism (via the Lorentz force) within brain signaling. It means that EM ToC offer a plausible physics mechanism linking consciousness to brain causality.
However, even with advantages like this, and like other ToC that identify the informational aspects of neuronal circuits as correlates of consciousness, structures of CNS EM, as an explanation of the origins of consciousness, similarly leaves an explanatory gap (where magical emergence comes in) for how consciousness is generated by EM phenomena. But EM has an aspect that gives it an explanatory future otherwise apparently lacking. Unlike computational ToC, an EM ToC is grounded in the fundamental physics of brain activity.
Even without any claims about which aspects or scales of EM might be relevant for how EM phenomena generate consciousness, it is clear that an EM ToC introduces a significant adjustment to ideas of “substrate independence.” An EM ToC claims that consciousness is substrate dependant. Only a substrate of EM fields of the kind expressed by the brain will do the job. Contrast this with a general-purpose computer running software of any kind. The EM field system physics that is a general-purpose computer need have no direct relationship with the EM field system physics of the brain. In the case of the general-purpose computer (regardless of whether it is claimed conscious or not) the EM fields comprising the general-purpose computer can be organized in any way that is consistent with the execution of the software it hosts (from an abacus to a steam computer). The EM basis of the substrate of a general-purpose computer is radically and irreconcilably different to that of the brain. Note that an (inorganic) artificially originated consciousness based on an EM ToC, for example, requires chip components that generate the same EM phenomena that brain cells generate – at the same spatial and temporal scales. That kind of physics replication activity is, so far, completely missing from the set of options used by neuroscience. It would operate with the same EM field substrate as the natural (organic) brain. The interesting potential future that this suggests is one where the equivalence of a brain and a general-purpose computer can be conclusively scientifically tested based on the idea of substrate dependence introduced by an EM ToC.
Other Ways of Getting Consciousness Into Computations
If strong emergence is not considered to be a satisfactory explanation of how consciousness arises, then a reasonable alternative might be that, rather than it emerging at some point in a complex system, it was actually present all along – perhaps even as an exotic field or particle or similar component that comprises the fundamental fabric of the universe. Some ToC include a proposal that consciousness in some most elemental or fundamental form, is a currently unrecognized (in that it is missing from the standard model of particle physics) basic constituent of the universe. For example Benjamin Libet’s “conscious mental field (CMF)” that “ would not be in any category of known physical fields, such as electromagnetic, gravitational, etc. ” ( Libet, 1994 ). Such proposals recognize that in a more comprehensive appreciation of the nature of the universe’s most basic composition we would appreciate consciousness in the same way that we appreciate that the fundamental constituents of the universe we know about have properties such as mass and spin and charge. Variations of this idea either propose that everything is, to some degree, consciousness [panpsychism ( Skrbina, 2007 ; Goff et al., 2018 )] or that consciousness emerges in a recognizable form, or reaches a critical threshold, only under certain constructions. Clearly, brains would be one such construction (indeed currently the only such construction known to us), but even then, there needs to be an explanation of why some aspects of nervous system function have consciousness and why some have not.
The Integrated Information Theory (IIT) ToC, another member of this class of ToC, seeks to find an informational criterion (such as the extent to which information is integrated) to define the presence or the amount of consciousness that certain constructions (biological or otherwise) will possess ( Balduzzi and Tononi, 2008 ; Tononi, 2008 ; Oizumi et al., 2014 ; Tononi et al., 2016 ). To ground the information transformations in consciousness, it has been proposed that all information carries with it, or inherits, or is formed from, a most basic and indivisible mote of consciousness, which is, again, implicitly posed as an undiscovered member or property of an upgraded standard model of particle physics (although it is not presented in standard-model terms). The desire to bring information into the fold of fundamental physics is a topic of exploration within physics more broadly ( Walker et al., 2017 ). We note in passing that an interesting connection between IIT and EM fields has been posed twice to date ( Barrett, 2014 ; McFadden, 2020 ). This may offer IIT a future as an EM field ToC.
Rather than start a ToC that implicitly relies on an undiscovered fundamental entity and engage in implementing whatever radical changes to the standard model are necessitated by it, EM ToC start with and are located within the relevant quadrant of the existing standard model of particle physics. We already know standard-model EM field properties naturally satisfy the necessary basic requirements of an originator of consciousness. The EM fields are large in spatial extent: the electric and magnetic fields of the brain pervade the entire space occupied by the brain, extending out into the space outside it. The EM fields are impressed on space in exquisite detail consistent with the detail we experience in perceptual fields (such as vision). The EM fields originate at the scale of the membrane in thousands of square meters of a huge electric field spanning the 5 nm membrane enclosure of all neurons and astrocytes. This forms the basis of (a kind of blank canvas for) a nested dynamic hierarchical organizational EM field structure with seven or eight orders of magnitude of spatial detail, extending to the cm scale. The endogenous EM fields of the brain are intense in that they dominate, in a signal strength sense, all the underlying chemical “EM noise” produced by the atomic-level structures generating it in its total form (on a scale that systematically influences its own neuronal excitability – see the above notes on ephaptic transmission).
The EM fields are intrinsically unified: the electric and magnetic fields of the brain are each a single object and inexorably present and modulated when any neural activity occurs. This unification provides a natural route to a solution to another well-known but unexplained property of consciousness: it’s striking and seamless unification of all the experiential modes of vision, audition, touch, olfaction and gustation, along with all the emotions ( Cleeremans and Frith, 2003 ; Bayne, 2010 ). Natural field superposition also solves the “combination” problem where emergent “wholes,” of a qualitatively unique character can be traced back to its vectorially superadded EM field parts. It facilitates the transformation to weak emergence discussed above. Contents of consciousness delivered by EM fields can enter consciousness merely through the seamless natural integrative superposition (a vector field property) of new field contributions produced by the underlying neural activity originating it. The EM field is also a parsimonious solution to problems related to time, for example, the need for a mechanism that explains how contents of consciousness delivered by EM fields can arrive and leave at the temporal rate and temporal resolution we observe and with the temporal continuity and discontinuity we observe. EM fields have the potential to provide that mechanism. We have already addressed the issue of causality that EM fields uniquely address in well-known physics terms. We will shortly discuss how EM fields naturally possess a potential to address the “symbol grounding/binding” issue. These issues have a long history of prominence in the science of consciousness ( Harnad, 1990 ; Treisman, 1996 ; Revonsuo and Newman, 1999 ; Roskies, 1999 ; Singer, 2001 ; Chalmers, 2016 ; Kent and Wittmann, 2021 ), and EM fields seem naturally suited to potentially offer a solution to them. At least, there is no aspect of these phenomena that seems obviously beyond the scope of EM fields.
However, despite the suitability of EM to potentially account for longstanding, nuanced and unexplained aspects of consciousness, yet again we arrive at the fact that the thing that is missing from an EM field account of consciousness is the troublesome aspect of its delivery: by “being the EM fields.” But this, we hold, is actually our problem , not a problem for nature. We are the ones that have failed to bring a 1st-person perspective into fundamental physics. The existing standard model of particle physics is empty of all content specifying “what it is like to be” any of the multitude of standard model entities (of “being” a muon or a neutrino or an EM field, for example). The neuroscience of consciousness, and its novel explanandum, have proved (albeit inadvertently) that the EM fields, a standard model entity, can originate a 1PP. Perhaps this deep and persistent evidence anomaly will motivate some attention by physicists to its standard model. It seems that one way or another, the standard model is up for an eventual makeover to formally introduce the 1PP to its otherwise prodigious predictive capacities. This reinforces the need for a future neuroscience/physics collaboration in the science of consciousness. Meanwhile, the recommended low hanging fruit of a convergence on EM fields is good preparation for it.
The Simulation Grounding Problem
As well as respecting the fine structure and function of the nervous system components, EM ToC naturally offers neuroscientists the potential to address the symbol binding problem (an issue brain science inherited by adopting paradigms from computer science). “Grounding,” in the sense of models of brain or cognitive function, can take on different definitions ( Harnad, 1990 ). Grounding addresses the sense in which symbols can be regarded as having a reliable relationship with the external environmental inputs that evoke the symbol (or other symbolic representation, such as the distributed activation states in an artificial neural network) or with the outputs to the external environment. A simple thermostat can be said to be grounded in this sense, but not (panpsychism excepted) in the sense that there is any meaning to its operation other than the interpretation of its input, output, and setpoint values in a more comprehensive context, such as in the humans employing or examining its structure and function. Symbols in more complex information processing contexts can stand for abstracted properties of the information. In these cases the complexity comes about by, for example, processing large quantities of information, combining it with previously acquired information, and being directed by explicit or generic objectives and so forth. This permits the analogy of these complex information processes with cognitive functions. Invariably, these information processing models are implemented on digital computers.
Variability in the definitions of grounding is presumably a large part of why it is claimed that the symbol grounding problem has been solved, hasn’t been (but could be) solved, can’t be solved, or isn’t a problem ( Taddeo and Floridi, 2005 ; Steels et al., 2007 ; Cubek et al., 2015 ). When considering how consciousness arises, we recognize that various cognitive processes are associated with very distinct and stable conscious states (experiences). These experiences literally are the symbol that becomes bound to brain events. Not only do our cognitions produce experienced conscious states (e.g., frustration, excitement, thirst, redness, fatigue, boredom, anger and so forth), but we are not the least bit unaware of their meaning – we don’t confuse feeling hungry with feeling short of breath; we are not confused about why these conscious states come and go because we are not observing or witnessing them, we are those states. The question of grounding in this context is how does the flow of information from interoceptive and exteroceptive systems give rise to the neural activity that generates these utterly familiar and innately interpretable experiences? Neuroscientists would agree that the brain activity occasioned by those inputs, in interaction with the states of the relevant brain regions when receiving the inputs, would dictate the particular quality of the conscious experience. But neuroscientists would likely be very reluctant to say that such states represented in the brain’s activity are grounded by the fact that the signals arise from (for example) vagal afferents from the viscera – if that was true it would not be possible to evoke sensations by stimulation higher up the pathway, and while direct brain stimulation is a very crude and unrealistic substitute for the precise and intricate patterns of activations that occur physiologically, stimulation of the cerebral cortex in awake people can still give rise to conscious experiences appropriate to the modalities known to be present in those cortical regions ( Raccah et al., 2021 ). Phantom limb pathologies also attest to the centrality of cranial brain matter in originating the kind and degree of experiences, resulting in perceptual “grounding” in symbols applied to externalities that do not exist ( Giummarra et al., 2007 ).
If it is held that the origins of meaning can’t be found in the ambient energies in the environment that construe adequate stimuli for sensors, and it is also granted that the inherent meaningfulness of conscious experiences means they must be considered to be grounded, it could be proposed that only consciousness can ground representations expressed in brain activity. For neuroscientists engaged in an EM ToC, this means the basis of grounding is intrinsically there to be found in the activity of the brain’s signaling physics itself – specifically those aspects of its function that are not those abstracted as the signals for information processes in computational models. This makes a sharp distinction between EM ToC and computational theories: the former claims that the crucial fundamental physics mechanisms are the very phenomena that computational theories discard as irrelevant.
What is proposed here isn’t an EM ToC but a case for why a ToC should be sought in the EM phenomena of brains. It proposes EM as the answer to the challenge: “ Which electrical property provides the most fruitful explanatory basis for understanding consciousness remains an open question ” ( Wu, 2018 ). In the process we find that neuroscience mixed with EM physics locates the center of the study of consciousness. Engaging this possibility, for neuroscientists, means bringing an end to a long era of abstracting-away EM phenomena. Neuroscientists will be required to embrace fundamental physics at a new level of complexity. Neuroscience and physics communities, connected in a joint need to resolve a troublesome and novel explanandum, are likely to be required to accommodate each other’s needs. What the standard model of particle physics might look like after this project is completed, we can only guess at.
Why then, would EM ToC offer an incentive for more neuroscientists to engage with consciousness? The primary reason is that EM fields are the fundamental physics of neurons and glia in the brain. It literally manifests the computations, or signal processing, or information processing/integration activities performed by connected ensembles of cells that we know generate a 1st-person perspective. An EM ToC also has built-in, natural routes to solutions to the thorniest issues of consciousness such as time, unity, binding, combination and causality. Most importantly, it provides the possibility of identifying the outward signs of a mechanism in the normal fundamental terms of EM field physics, as opposed to merely describing the correlates of our mental abstractions of it. A focus on an EM field basis for consciousness does not in any way diminish the role of computation in the operation of the nervous system. Nor does it invalidate any other existing theory of consciousness. Computational activity, or aspects of that activity, will define the particulars of conscious experience, but the computations are not what generates consciousness: that is a deeper level of the fundamental signaling physics originating in the activity of the membrane. That signaling is entirely and only an EM field phenomenon.
Author Contributions
Both authors listed have made a substantial, direct, and intellectual contribution to the work, and approved it for publication.
This work was supported by a seed grant from the University of Melbourne, Department of Anatomy and Physiology.
Conflict of Interest
The authors declare that the research was conducted in the absence of any commercial or financial relationships that could be construed as a potential conflict of interest.
Publisher’s Note
All claims expressed in this article are solely those of the authors and do not necessarily represent those of their affiliated organizations, or those of the publisher, the editors and the reviewers. Any product that may be evaluated in this article, or claim that may be made by its manufacturer, is not guaranteed or endorsed by the publisher.
- ^ For example, Computational Models of Cognition, Center for Brains Minds + Machines: Summer Course 2018. See https://www.youtube.com/watch?v=TFyAEHk5asY .
Augustine, G. J., and Kasai, H. (2007). Bernard Katz, quantal transmitter release and the foundations of presynaptic physiology. J. Physiol. 578, 623–625. doi: 10.1113/jphysiol.2006.123224
PubMed Abstract | CrossRef Full Text | Google Scholar
Balduzzi, D., and Tononi, G. (2008). Integrated information in discrete dynamical systems: motivation and theoretical framework. PLoS Comput. Biol. 4:e1000091. doi: 10.1371/journal.pcbi.1000091
Barrett, A. (2014). An integration of integrated information theory with fundamental physics. Front. Psychol. 5:63. doi: 10.3389/fpsyg.2014.00063
Bayne, T. (2010). The Unity of Consciousness. Oxford: Oxford University Press.
Google Scholar
Bedau, M. A. (1997). “Weak emergence,” in Philosophical Perspectives: Mind, Causation, and World , ed. J. Tomberlin (Malden, MA: Blackwell), 375–399. doi: 10.1111/0029-4624.31.s11.17
CrossRef Full Text | Google Scholar
Chalmers, D. (1997). “Facing up to the hard problem of consciousness,” in Explaining Consciousness: The Hard Problem , ed. J. Shear (Cambridge: MIT Press), 9–32.
Chalmers, D. J. (1995). Facing up to the problem of consciousness. J. Conscious. Stud. 2, 200–219.
Chalmers, D. J. (1996). The Conscious Mind: In Search of a Fundamental Theory. New York, NY: Oxford University Press.
Chalmers, D. J. (2006). “Strong and weak emergence,” in The Re-emergence of Emergence: the Emergentist Hypothesis From Science to Religion , eds P. Clayton and P. Davies (New York, NY: Oxford University Press), 244–254. doi: 10.1093/acprof:oso/9780199544318.003.0011
Chalmers, D. (2016). The combination problem for panpsychism. In Panpsychism: Contemporary Perspectives, G. Bruntrup, and L. Jaskolla, eds. (Oxford University Press), pp. 179-214.
Chiang, C.-C., Shivacharan, R. S., Wei, X., Gonzalez-Reyes, L. E., and Durand, D. M. (2019). Slow periodic activity in the longitudinal hippocampal slice can self-propagate non-synaptically by a mechanism consistent with ephaptic coupling. J. Physiol. 597, 249–269. doi: 10.1113/JP276904
Cleeremans, A., and Frith, C. (eds) (2003). The Unity of Consciousness. Oxford: Oxford University Press.
Constantine-Paton, M. (2008). Pioneers of cortical plasticity: six classic papers by Wiesel and Hubel. J. Neurophysiol. 99, 2741–2744. doi: 10.1152/jn.00061.2008
Cottingham, W. N., and Greenwood, D. A. (2007). An Introduction to the Standard Model of Particle Physics. Cambridge: Cambridge university press.
Crick, F., and Koch, C. (1990). Towards a neurobiological theory of consciousness. Semin. Neurosci. 2, 263–275.
Cubek, R., Ertel, W., and Palm, G. (2015). “A critical review on the symbol grounding problem as an issue of autonomous agents,” in KI 2015: Advances in Artificial Intelligence. KI 2015. Lecture Notes in Computer Science , Vol. 9324, eds S. Hölldobler, R. Peñaloza, and S. Rudolph (Cham: Springer), 256–263.
Eccles, J. C. (1982). The synapse: from electrical to chemical transmission. Annu. Rev. Neurosci. 5, 325–339. doi: 10.1146/annurev.ne.05.030182.001545
Giummarra, M. J., Gibson, S. J., Georgiou-Karistianis, N., and Bradshaw, J. L. (2007). Central mechanisms in phantom limb perception: the past, present and future. Brain Res. Rev. 54, 219–232. doi: 10.1016/j.brainresrev.2007.01.009
Glickstein, M. (2006). Golgi and Cajal: the neuron doctrine and the 100th anniversary of the 1906 Nobel Prize. Curr. Biol. 16, R147–R151. doi: 10.1016/j.cub.2006.02.053
Goff, P., Seager, W., and Allen-Hermanson, S. (2018). Panpsychism. The Stanford Encyclopedia of Philosophy. Available online at: https://plato.stanford.edu/entries/panpsychism/ (accessed on February 28, 2018).
Gregor, K., and LeCun, Y. (2010). Emergence of complex-like cells in a temporal product network with local receptive fields. arXiv [Preprint] Available online at: https://arxiv.org/abs/1006.0448
Harnad, S. (1990). The symbol grounding problem. Physica D 42, 335–346.
Jones, M. W. (2013). Electromagnetic-field theories of mind. J. Conscious. Stud. 20, 124–149.
Jones, M. W. (2017). Mounting evidence that minds are neural EM fields interacting with brains. J. Conscious. Stud. 24, 159–183.
Kelly, K. (1994). Out of Control: the Rise of Neo-biological Civilization. Reading, MA: Addison-Wesley.
Kent, L., and Wittmann, M. (2021). Time consciousness: the missing link in theories of consciousness. Neurosci. Conscious. 2021:niab011.
Koch, C. (2019). The Feeling of Life Itself: Why Consciousness is Widespread but Can’t be Computed. Cambridge, MA: MIT Press.
LeCun, Y., Bengio, Y., and Hinton, G. (2015). Deep learning. Nature 521, 436.
Levine, J. (1983). Materialism and qualia, the explanatory gap. Pac. Philos. Q. 64, 354–361. doi: 10.1111/j.1468-0114.1983.tb00207.x
Libet, B. (1994). A testable field theory of mind-brain interaction. J. Conscious. Stud. 1, 119–126. doi: 10.3389/fnhum.2012.00147
Linsker, R. (1986a). From basic network principles to neural architecture: emergence of orientation-selective cells. Proc. Natl. Acad. Sci. U.S.A. 83, 8390–8394. doi: 10.1073/pnas.83.21.8390
Linsker, R. (1986b). From basic network principles to neural architecture: emergence of orientation columns. Proc. Natl. Acad. Sci. U.S.A. 83, 8779–8783. doi: 10.1073/pnas.83.22.8779
Linsker, R. (1986c). From basic network principles to neural architecture: emergence of spatial-opponent cells. Proc. Natl. Acad. Sci. U.S.A. 83, 7508–7512. doi: 10.1073/pnas.83.19.7508
Martin, K. A. C. (2006). Where are the switches on this thing? Nature 440, 1113–1114.
McComas, A. (2011). Galvani’s Spark: The Story of the Nerve Impulse. Oxford: Oxford University Press.
McCulloch, W. S. (1965). Embodiments of Mind. Cambridge, MA: MIT Press.
McFadden, J. (2020). Integrating information in the brain’s EM field: the cemi field theory of consciousness. Neurosci. Conscious. 2020:13. doi: 10.1093/nc/niaa016
O’Connor, T. (2020). “Emergent properties,” in The Stanford Encyclopedia of Philosophy , ed. E. N. Zalta (Stanford, CA: Stanford University).
Oizumi, M., Albantakis, L., and Tononi, G. (2014). From the phenomenology to the mechanisms of consciousness: integrated information theory 3.0. PLoS Comput. Biol. 10:e1003588.
Olshausen, B. A., and Field, D. J. (1996). Emergence of simple-cell receptive field properties by learning a sparse code for natural images. Nature 381, 607–609. doi: 10.1038/381607a0
O’Shea, K., and Nash, R. (2015). An introduction to convolutional neural networks. arXiv [Preprint] Available online at: https://arxiv.org/abs/1511.08458
Pockett, S. (2013). Field theories of consciousness. Scholarpedia 8:4951. doi: 10.4249/scholarpedia.4951
Raccah, O., Block, N., and Fox, K. C. R. (2021). Does the prefrontal cortex play an essential role in consciousness? Insights from intracranial electrical stimulation of the human brain. J. Neurosci. 41, 2076–2087. doi: 10.1523/JNEUROSCI.1141-20.2020
Revonsuo, A., and Newman, J. (1999). Binding and consciousness. Conscious. Cogn. 8, 123–127. doi: 10.1006/ccog.1999.0393
Reyes, A. D. (2019). A breakthrough method that became vital to neuroscience. Nature 575, 38–39. doi: 10.1038/d41586-019-02836-6
Rich, J. (2010). Fundamentals of Cosmology. Berlin: Springer-Verlag.
Roskies, A. L. (1999). The binding problem. Neuron 24, 7–9.
Schmidhuber, J. (2015). Deep learning in neural networks: an overview. Neural Netw. 61, 85–117. doi: 10.1016/j.neunet.2014.09.003
Seth, A. (2009). Explanatory correlates of consciousness: theoretical and computational challenges. Cogn. Comput. 1, 50–63. doi: 10.1007/s12559-009-9007-x
Seth, A. (2021). Being You: A New Science of Consciousness. New York, NY: Penguin Publishing Group.
Singer, W. (2001). “Consciousness and the binding problem,” in Cajal and Consciousness - Scientific Approaches to Consciousness on the Centennial of Ramon Y Cajal’s Textura , ed. P. C. Marijuan (New York, NY: Annals of the New York Academy of Science), 123–146.
Skrbina, D. (2007). “Panpsychism,” in Internet Encyclopedia of Philosophy , (Cambridge, MA: MIT Press).
Steels, L., Loetzsch, M., and Spranger, M. (2007). Semiotic dynamics solves the symbol grounding problem. Nat. Prec. 1–15. doi: 10.1038/npre.2007.1234.1
Taddeo, M., and Floridi, L. (2005). Solving the symbol grounding problem: a critical review of fifteen years of research. J. Exp. Theor. Artif. Intell. 17, 419–445. doi: 10.1080/09528130500284053
Tononi, G. (2008). Consciousness as integrated information: a provisional manifesto. Biol. Bull. 215, 216–242.
Tononi, G., Boly, M., Massimini, M., and Koch, C. (2016). Integrated information theory: from consciousness to its physical substrate. Nat. Rev. Neurosci. 17:450.
Treisman, A. (1996). The binding problem. Curr. Opin. Neurobiol. 6, 171–178.
Valenstein, E. S. (2002). The discovery of chemical neurotransmitters. Brain Cogn. 49, 73–95. doi: 10.1006/brcg.2001.1487
Van Gulick, R. (2018). “Consciousness,” in The Stanford Encyclopedia of Philosophy Spring 2018 Edition , ed. E. N. Zalta (Berlin: Springer).
Walker, S. I., Davies, P. C. W., and Ellis, G. F. R. (2017). From Matter to Life: Information and Causality. Cambridge: Cambridge University Press.
Wallace, B. A. (2000). The Taboo of Subjectivity: Toward a New Science of Consciousness. New York, NY: Oxford University Press.
Wu, W. (2018). “The neuroscience of consciousness,” in The Stanford Encyclopedia of Philosophy , ed. E. N. Zalta (Stanford, CA: Stanford University).
Wurtz, R. H. (2009). Recounting the impact of Hubel and Wiesel. J. Physiol. 587, 2817–2823. doi: 10.1113/jphysiol.2009.170209
Keywords : consciousness, electromagnetism, information, neural signals, computation
Citation: Kitchener PD and Hales CG (2022) What Neuroscientists Think, and Don’t Think, About Consciousness. Front. Hum. Neurosci. 16:767612. doi: 10.3389/fnhum.2022.767612
Received: 31 August 2021; Accepted: 19 January 2022; Published: 24 February 2022.
Reviewed by:
Copyright © 2022 Kitchener and Hales. This is an open-access article distributed under the terms of the Creative Commons Attribution License (CC BY) . The use, distribution or reproduction in other forums is permitted, provided the original author(s) and the copyright owner(s) are credited and that the original publication in this journal is cited, in accordance with accepted academic practice. No use, distribution or reproduction is permitted which does not comply with these terms.
*Correspondence: Peter D. Kitchener, [email protected]
† These authors have contributed equally to this work
This article is part of the Research Topic
Electromagnetic Field Theories of Consciousness: Opportunities and Obstacles

The crystallization of memory: Study reveals how practice forms new memory pathways in the brain
A new study led by UCLA Health has shown that repetitive practice not only is helpful in improving skills but also leads to profound changes in the brain's memory pathways.
The research, published in the journal Nature and co-led by Rockefeller University, sought to unravel how the brain's ability to retain and process information, known as working memory, improves through training.
To test this, researchers tasked mice with identifying and recalling a sequence of odors over the course of two weeks. Researchers then tracked neural activity in the animals as they practiced the task by using a novel, custom-built microscope that can image cellular activity in up to 73,000 neurons simultaneously throughout the cortex.
The study revealed a transformation in the working memory circuits located in the secondary motor cortex as the mice repeated the task through time. As the mice were first learning the task, the memory representations were unstable. However, after repeatedly practicing the task, the memory patterns began to solidify or "crystalize," said corresponding author and UCLA Health neurologist Dr. Peyman Golshani.
"If one imagines that each neuron in the brain is sounding a different note, the melody that the brain is generating when it is doing the task was changing from day to day, but then became more and more refined and similar as animals kept practicing the task," Golshani said.
These changes give insights into why performance becomes more accurate and automatic following repetitive practice.
"This insight not only advances our understanding of learning and memory but also has implications for addressing memory-related disorders," Golshani said.
The work was performed by Dr. Arash Bellafard, project scientist at UCLA in close collaboration with Dr. Alipasha Vaziri's group at Rockefeller University.
- Intelligence
- Neuroscience
- Learning Disorders
- Educational Psychology
- Brain Injury
- Memory-prediction framework
- Social cognition
- Limbic system
- Deep brain stimulation
- Asperger syndrome
Story Source:
Materials provided by University of California - Los Angeles Health Sciences . Original written by Will Houston. Note: Content may be edited for style and length.
Journal Reference :
- Arash Bellafard, Ghazal Namvar, Jonathan C. Kao, Alipasha Vaziri, Peyman Golshani. Volatile working memory representations crystallize with practice . Nature , 2024; DOI: 10.1038/s41586-024-07425-w
Cite This Page :
Explore More
- Autonomous Drones With Animal-Like 'Brains'
- How Practice Forms New Memory Pathways
- Reversing Brain Damage Caused by Ischemic Stroke
- Earth-Sized Planet Orbiting Ultra-Cool Dwarf
- Robots' Sense of Touch as Fast as Humans?
- Avian Flu Detected in NYC Wild Birds
- Metro-Area Quantum Computer Network Demo
- Iconic Baobab Tree's Origin Story
- 'Warm-Blooded' Dinos: 180 Million Years Ago
- Reaching 1,000 Degrees C With Solar Power
Trending Topics
Strange & offbeat.
Advances in Cognitive Research, Artificial Intelligence and Neuroinformatics
Proceedings of the 9th International Conference on Cognitive Sciences, Intercognsci-2020, October 10-16, 2020, Moscow, Russia
- Conference proceedings
- © 2021
- Boris M. Velichkovsky 0 ,
- Pavel M. Balaban 1 ,
- Vadim L. Ushakov 2
National Research Center "Kurchatov Institute", Moscow, Russia
You can also search for this editor in PubMed Google Scholar
Institute of Higher Nervous Activity RAS, Moscow, Russia
Institute for advanced brain studies, lomonosov moscow state university, moscow, russia.
- Offers an overview of empirical methods and theoretical models in cognitive science
- Merges perspectives from philosophy of mind, psychology, neuroscience, artificial intelligence, and robotics
- Unique snapshot of neurocognitive research and applications in the northeast of Europe
Part of the book series: Advances in Intelligent Systems and Computing (AISC, volume 1358)
Included in the following conference series:
- Intercognsci: International Conference on Cognitive Sciences
Conference proceedings info: Intercognsci 2020.
60k Accesses
63 Citations
14 Altmetric
This is a preview of subscription content, log in via an institution to check access.
Access this book
- Available as EPUB and PDF
- Read on any device
- Instant download
- Own it forever
- Compact, lightweight edition
- Dispatched in 3 to 5 business days
- Free shipping worldwide - see info
Tax calculation will be finalised at checkout
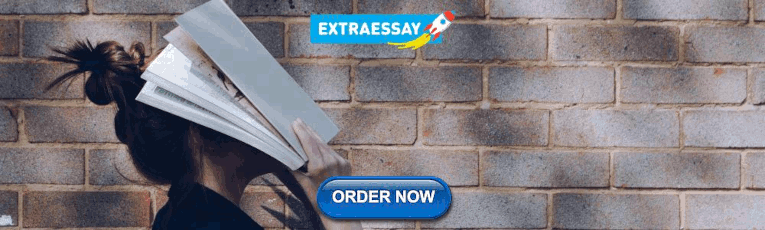
Other ways to access
Licence this eBook for your library
Institutional subscriptions
Table of contents (84 papers)
Front matter, conference invited lectures, language, cognitive systems, and the methodology of observation.
- Andrej A. Kibrik
Trends and Perspectives in Cognitive Research
Boris M. Velichkovsky
Cognitive Development, Skills, and Aging
Theoretical and empirical criteria for selecting cognitive over-performers: data from a primary school in moscow.
- Elena Khoroshkova, Valeria Sizova, Anastasiia Liashenko, Marie Arsalidou
Perceptual-Cognitive Demands of Esports and Team Sports: A Comparative Study
- Alyona Grushko, Olga Morozova, Mikhail Ostapchuk, Ekaterina Korobeynikova
The Relation Between Cognitive Flexibility and Language Production in Preschool Children
- Ekaterina Oshchepkova, Daria Bukhalenkova, Aleksander Veraksa
A Digital Psychophysiological Mapping of Primary School Children with/without Learning Disabilities
- Lyudmila V. Savchuk, Sofia A. Polevaya, Kirill N. Gromov, Alexander I. Fedotchev, Sergey B. Parin, Oksana V. Balandina et al.
Theory of Mind and Behavioral Control in Children with and without Borderline Intellectual Functioning
- Galina A. Vilenskaya, Evgenya I. Lebedeva
Bilingualism and Reserve: Etiology of Successful Aging
- Federico Gallo, Andriy Myachykov, Yury Shtyrov
Perception, Memory, and Higher Cognition
Recognition of visual stimuli which proceeded by socially significant images: an erp study.
- Natalia Gerasimenko, Anastasiia Kushnir, Elena Mikhailova
How Areas of Ventral Visual Stream Interact When We Memorize Color and Shape Information
- Stanislav Kozlovskiy, Anton Rogachev
The Effectiveness of Metacognitive Hints in Insight Problem Solving
- Sergey Korovkin, Anna Savinova
Effects of Online Repetitive Transcranial Magnetic Stimulation on the Frequency of Insights During Anagram Solving
- Alexandra G. Poydasheva, Ilya S. Bakulin, Dmitry Yu. Lagoda, Alexei A. Medyntsev, Dmitry O. Sinitsyn, Petr N. Kopnin et al.
The Mirror Neuron System Activity is Higher with Personal Direct Interaction
- Ekaterina Karimova, Sabir Burkitbaev, Nikita Katermin
Gender Differences in Object and Spatial Inattentional Blindness Under Working Memory Load
- Boris B. Velichkovsky, Sofia Popova
Effect of ‘Dry’ Immersion on Visual Illusions
- Inna Sosnina, Vsevolod Lyakhovetskii, Konstantin Zelenskiy, Elena Tomilovskaya, Valeria Karpinskaya
Auditory Mechanisms for Analyzing Conspecific Movement
- Vyacheslav A. Orlov, Vadim L. Ushakov, Irina G. Andreeva
Other volumes
- Neurophysiology of cognitive processes
- Sensory-perceptual processes
- Visual perception
- Cognitive processing
- Functional brain connectivity
- Eye movements research
- Ambiguity Resolution
- Effective connectivity
- Neural correlates of consciousness
- Molecular mechanisms of cognitive abilities
- Language mapping
- Execution of Movements
- Cognitive Architecture
- Human-Robot communication
- Affective Robots
- Automatic emotion recognition
- Eye and Brain Computer Interfaces
- Intercognsci 2020
About this book
This book reports on theoretical and experimental research answering key questions in neuroscience, philosophy of mind, and cognitive research. It gives a special emphasis on findings achieved within the territory of the former U.S.S.R, which has remained largely unknown to an international readership. The volume gathers authoritative studies on cognitive development, consciousness, attention and perception. It covers research on eye movements, language, speech and semantics, emotion, as well as brain functional states, and a variety of decision-making processes. It also highlights important advances in cognitive robotics and artificial intelligence, discussing brain-computer interfaces and other practically-relevant technologies. It includes studies on human subjects, in both healthy and disease conditions, and investigations on the molecular mechanisms of cognition in animal models.
Chapters are based on invited lectures and peer-reviewed contributions to the 9th International Conference on Cognitive Sciences, Intercognsci–2020, held on October 10-16, 2020, in Moscow. The conference was organized by the Interregional Association of Cognitive Studies, with the participation of the Pavlov Society for Neurophysiology and Higher Nervous Activity, and supported by the Russian Academy of Sciences, the Russian Foundation for Basic Research and a number of the north eastern European research institutions. All in all, this book provides cognitive scientists around the world with a timely snapshot of interdisciplinary research and cutting-edge models, and a major source of inspiration for future collaborations in the areas of artificial intelligence and cognitive neuroscience.
Editors and Affiliations
National research center "kurchatov institute", moscow, russia.
Pavel M. Balaban
Vadim L. Ushakov
Bibliographic Information
Book Title : Advances in Cognitive Research, Artificial Intelligence and Neuroinformatics
Book Subtitle : Proceedings of the 9th International Conference on Cognitive Sciences, Intercognsci-2020, October 10-16, 2020, Moscow, Russia
Editors : Boris M. Velichkovsky, Pavel M. Balaban, Vadim L. Ushakov
Series Title : Advances in Intelligent Systems and Computing
DOI : https://doi.org/10.1007/978-3-030-71637-0
Publisher : Springer Cham
eBook Packages : Intelligent Technologies and Robotics , Intelligent Technologies and Robotics (R0)
Copyright Information : The Editor(s) (if applicable) and The Author(s), under exclusive license to Springer Nature Switzerland AG 2021
Softcover ISBN : 978-3-030-71636-3 Published: 18 April 2021
eBook ISBN : 978-3-030-71637-0 Published: 17 April 2021
Series ISSN : 2194-5357
Series E-ISSN : 2194-5365
Edition Number : 1
Number of Pages : XIX, 740
Number of Illustrations : 70 b/w illustrations, 117 illustrations in colour
Topics : Computational Intelligence , Machine Learning , Biomedical Engineering and Bioengineering , Cognitive Psychology
- Publish with us
Policies and ethics
- Find a journal
- Track your research
Color as an important biological variable in zebrafish models: Implications for translational neurobehavioral research
Affiliations.
- 1 Bioscience Institute, University of Passo Fundo (UPF), Passo Fundo, RS, Brazil; The International Zebrafish Neuroscience Research Consortium (ZNRC), Slidell, LA, USA; Moscow Institute of Physics and Technology, Moscow, Russia. Electronic address: [email protected].
- 2 Bioscience Institute, University of Passo Fundo (UPF), Passo Fundo, RS, Brazil; Postgraduate Program in Environmental Sciences, University of Passo Fundo (UPF), Passo Fundo, Brazil.
- 3 Bioscience Institute, University of Passo Fundo (UPF), Passo Fundo, RS, Brazil.
- 4 Almazov Medical Research Center, Ministry of Healthcare of Russian Federation, St. Petersburg, Russia; Institute of Translational Biomedicine, St. Petersburg State University, St. Petersburg, Russia; Neuroscience Program, Sirius University, Sochi, Russia.
- 5 Granov Russian Research Center of Radiology and Surgical Technologies, St. Petersburg, Russia; Moscow Institute of Physics and Technology, Moscow, Russia; Neuroscience Program, Sirius University, Sochi, Russia.
- 6 Sechenov First Moscow State Medical University, Moscow, Russia; Maastricht University, Maastricht, the Netherlands; Research Institute of General Pathology and Pathophysiology, Moscow, Russia.
- 7 Scientific Research Institute of Neurosciences and Medicine, Novosibirsk, Russia; Zelman Institute of Medicine and Psychology, Novosibirsk State University, Novosibirsk, Russia.
- 8 Ural Federal University, Ekaterinburg, Russia; School of Pharmacy, Southwest University, Chongqing, China. Electronic address: [email protected].
- PMID: 33359096
- DOI: 10.1016/j.neubiorev.2020.12.014
Color is an important environmental factor that in multiple ways affects human and animal behavior and physiology. Widely used in neuroscience research, various experimental (animal) models may help improve our understanding of how different colors impact brain and behavioral processes. Complementing laboratory rodents, the zebrafish (Danio rerio) is rapidly emerging as an important novel model species to explore complex neurobehavioral processes. The growing utility of zebrafish in biomedicine makes it timely to consider the role of colors in their behavioral and physiological responses. Here, we summarize mounting evidence implicating colors as a critical variable in zebrafish models and neurobehavioral traits, with a particular relevance to CNS disease modeling, genetic and pharmacological modulation, as well as environmental enrichment and animal welfare. We also discuss the growing value of zebrafish models to study color neurobiology and color-related neurobehavioral phenomics, and outline future directions of research in this field.
Keywords: Animal model; Cognition; Drugs; Emotional behavior; Social phenotypes.
Copyright © 2020 Elsevier Ltd. All rights reserved.
Publication types
- Research Support, Non-U.S. Gov't
- Behavior, Animal*
- Disease Models, Animal
- Models, Animal
- Translational Research, Biomedical
Neuroscience study shows different brain reactions to music based on song familiarity
M usic is a fundamental part of human culture, experienced daily by people across the globe to evoke emotions and memories. A recent study published in Cerebral Cortex has now provided new insights into how our brains process music we know well compared to tunes that are new to us. Using advanced brain imaging techniques, researchers have found that familiar and unfamiliar music engage different memory systems in our brains, shedding light on the neurobiological processes underlying our musical experiences.
“Music is a cross-cultural and cross-racial medium for emotional expression and transmission, and it is an indispensable part of human life. However, what exactly happens in the brain when listening to music is not well understood. Therefore, we initiated this research to explore the differences in brain neural activity when listening to familiar versus unfamiliar music,” explained study author Li Qiang, an associate professor at Guizhou Education University.
To explore these questions, the researchers designed an experiment utilizing functional magnetic resonance imaging (fMRI), a technique that enables the visualization of active brain regions by detecting changes associated with blood flow. This method is particularly suited for studies like this as it allows for precise anatomical mapping of brain activity in response to specific stimuli—in this case, music.
The study involved 21 young adult participants, all of whom were non-musicians to avoid the confounding effects of musical training on brain activity patterns. These participants were exposed to 130 song excerpts while undergoing fMRI scans. The songs were carefully selected to cover a range of familiarity — from well-known pop hits to obscure tracks — ensuring a robust test of the brain’s response to familiar and unfamiliar music. Each participant rated the familiarity of each song using a five-point scale immediately after listening, providing data that correlated subjective experiences of familiarity with objective measurements of brain activity.
For familiar music, the researchers found pronounced activation in regions of the brain typically associated with explicit memory systems, including the hippocampus and frontal areas. These areas are crucial for recalling facts and personal experiences, suggesting that familiar songs trigger a recall of specific memories or associated emotions.
This result aligns with the theory that familiar melodies may help retrieve stored information about the context in which the music was heard, such as remembering the moment one first heard a favorite song or significant events tied to certain tunes.
In contrast, unfamiliar music was shown to activate regions associated with implicit memory, such as the basal ganglia. Implicit memory operates without conscious awareness and supports our ability to develop skills and habits. The activation of these areas suggests that hearing unfamiliar music engages the brain’s learning mechanisms, which may facilitate the subconscious integration of new auditory patterns and structures without immediate conscious recognition or recall.
Interestingly, the study highlighted that not only are different memory systems activated by familiar versus unfamiliar music, but these systems also interact differently with other neural networks across the brain. For instance, familiar music led to increased connectivity between memory-related regions and the auditory cortex, which processes sound. This suggests a more integrated and perhaps intensive processing network that links directly to stored memories and emotions, enhancing the emotional and mnemonic quality of the music listening experience.
On the other hand, unfamiliar music elicited less connectivity between these memory regions and the auditory cortex but involved more widespread activation across the brain. This pattern may reflect the brain’s attempt to understand and categorize new auditory information, engaging a broader network of regions to process and perhaps store new musical information for future reference.
“Listening to music is both simple and not simple,” Qiang told PsyPost. “It is simple because humans have the ability to appreciate music without any musical training. It is not simple because the process behind listening to music involves complex neural mechanisms. Listening to music of different familiarity activates two completely different memory systems; familiar music triggers the explicit music memory system, while unfamiliar music activates the implicit music memory system.”
One key limitation is the use of a homogenous participant group — primarily young adults — which might not represent the full variability of the general population. Additionally, the focus on pop music may not capture the neural responses to other music genres that could engage the brain differently.
Future research could expand by including diverse age groups, various musical training backgrounds, and different types of music to see if these findings hold universally. Moreover, researchers are interested in exploring the broader question of why humans are uniquely attuned to music and how this “regulated sound” becomes so deeply embedded in our culture and emotional experiences.
“Listening to and appreciating music is a very important capability, crucial for human empathy and the experience and expression of emotions,” Qiang noted. “We understand that in some countries and regions, parents believe that listening to music can affect their children’s studies and thus limit their exposure to music. However, we believe this might affect the development of the children’s music memory networks.”
“The long-term goal of this research is to solve a very fascinating question: how does music become music?” the researcher added. “We hear many sounds in our lives, but why is it that only music becomes ‘music’? What information is encoded in our genes that makes us particularly fascinated by this regulated sound, and what is this regulation? These are questions we want to answer throughout our lifetime.”
The study, “ Neural correlates of musical familiarity: a functional magnetic resonance study ,” was authored by Qiang Li, Guangyuan Liu, Yuan Zhang, Junhua Wu, and Rong Huang.
Our brains trick us into thinking consciousness can reside outside the body, new Northeastern research proves
- Search Search
Our brains trick us into thinking consciousness can reside outside the body, new Northeastern research says
In the Neuroscience of Consciousness, psychology professor Iris Berent argues that the debate stems from the delusional biases in the way humans think about the separation, or lack thereof, between body and mind.

- Copy Link Link Copied!
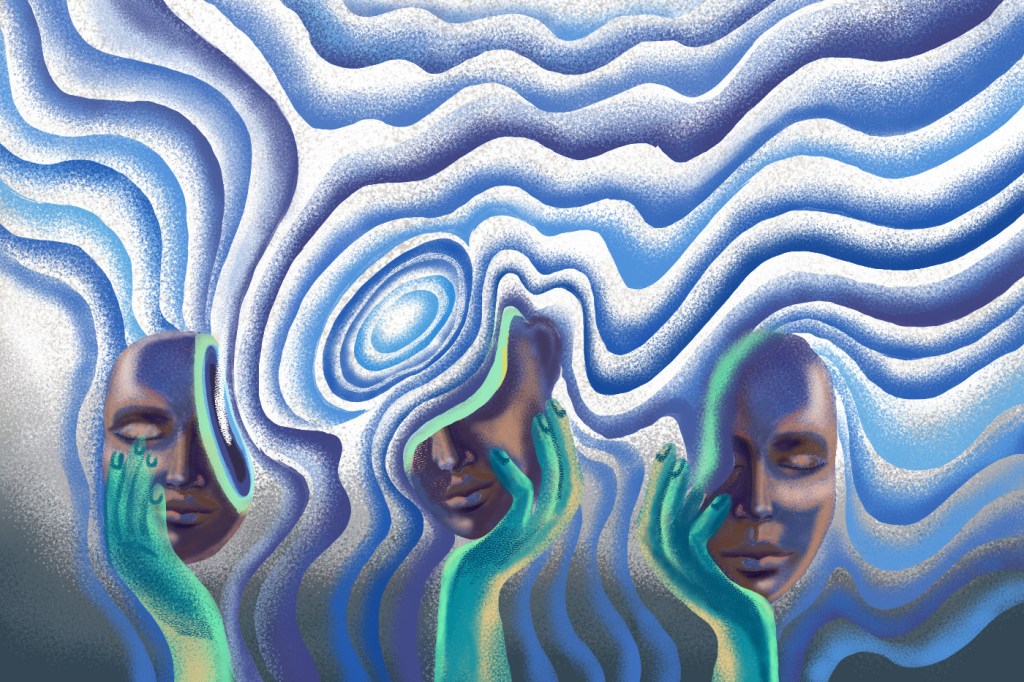
The origins of consciousness have been debated by philosophers for centuries.
Each conscious person has a sense of “being me,” which invariably gives rise to the question of where that sense originated — from within or outside the body, says Northeastern University psychology professor Iris Berent .
“How do human brains give rise to this experience? That’s the big mystery, right?” she says.
Influential philosopher David Chalmers famously won a bet in 2023 for claiming consciousness exists beyond the merely physical, Berent says.
But she says the question of where consciousness exists is a false one — and she has a new paper that presents her position.
In the Neuroscience of Consciousness journal she argues that the debate stems from the delusional — albeit natural — biases in the way humans think about the separation, or lack thereof, between body and mind.
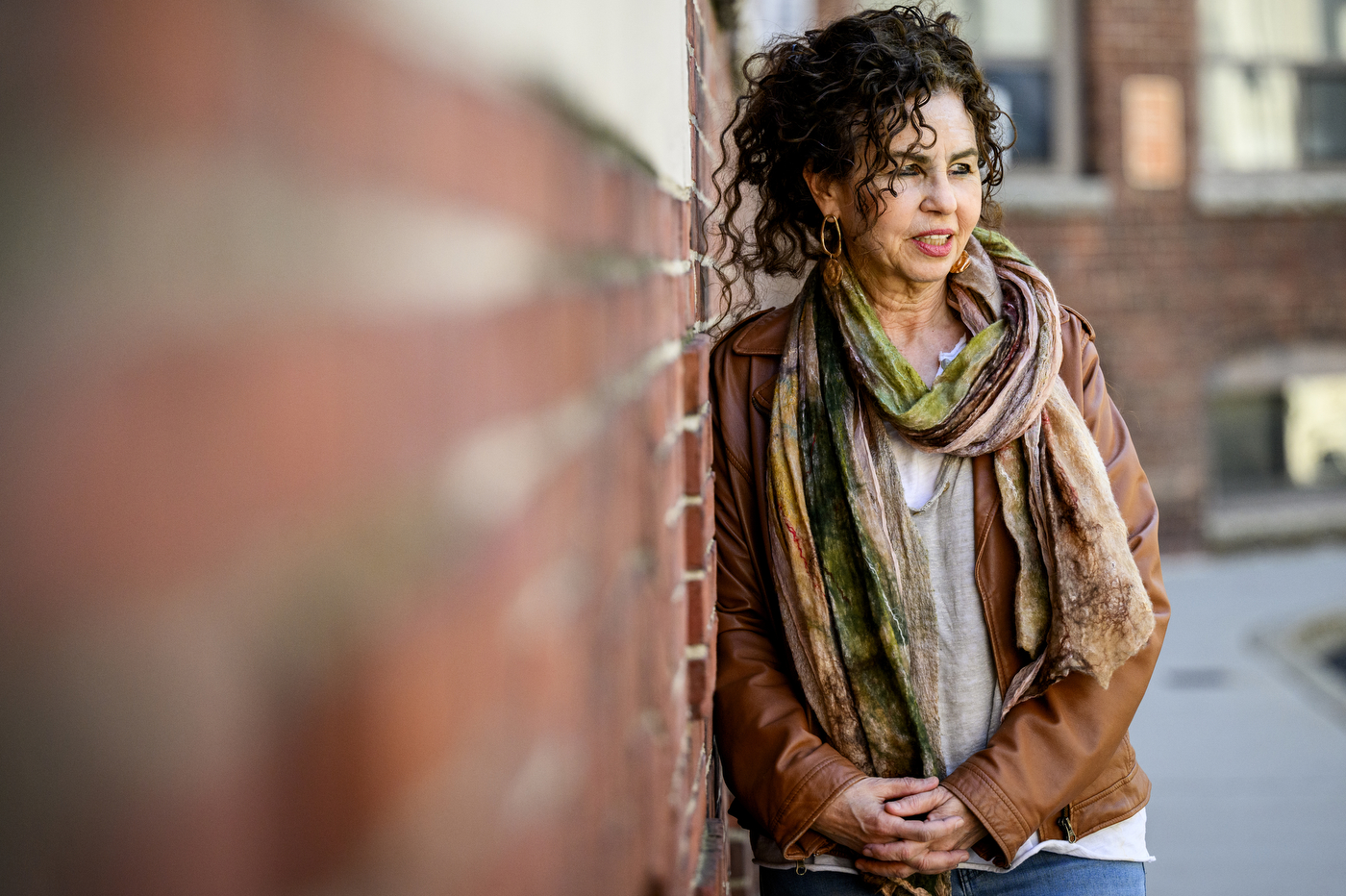
“One of the biases is dualism, intuitive dualism — the fact that we perceive minds as separate from our bodies.”
“The extent to which we look at consciousness and think that it is this really mysterious thing could very well arise from how we see it rather from what consciousness really is,” Berent says.
“Consciousness isn’t hard. Psychology is,” she says.
Mary and the zombie
Berent points to an experiment in perception she conducted in her lab that used the well-known Mary and the zombie hypothetical exercise, but with a twist.
According to the experiment, when people are asked to think of a zombie twin of themselves, they describe a creature with their physical features but without their thoughts or feelings.
“They intuit that the mind, consciousness included, is really separate from the physical,” Berent says.
In the other thought experiment, Mary is a neuroscientist who knows everything about color and how the brain perceives color, even though she lives in a black-and-white world.
When Mary sees a red rose for the first time, people participating in the experiment say she learns something outside the bounds of physical, scientific explanation.
Editor’s Picks
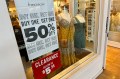
CPI report shows inflation eased in April, but consumer prices still rose 3.4% from a year ago
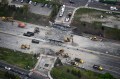
One-third of bridges in the United States need fixing. Here’s how to do that without wreaking havoc on supply chains and commuters
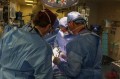
What are the risks involved with donating a kidney? A transplant expert explains why pig organs may be the only option for some
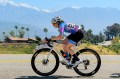
She’s a pro cyclist racing on weekends, but this Northeastern grad says co-ops put her business career in motion
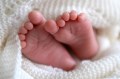
What’s in a name? A linguistics expert explains why some baby names dominate the charts year after year
Berent says she decided to challenge these conclusions with two additional questions.
“The first question is kind of a reality check question, which is do they think Mary’s case is significant? Is it transformative? And everybody said, ‘Sure, it’s super transformative,’” Berent says.
“We also asked how likely is it that this experience will actually show up in her brain? If we scan her brain, will it light up? And it turns out that that’s exactly what people said. It will significantly register in the brain.”
“The point being, in the condition of the zombie people say, ‘no,’ (consciousness) is not physical,” Berent says. “And in Mary’s condition, people say it’s physical.”
“If people change their mind in this way, it can’t possibly be that in reality consciousness has changed. It must be that there is something within the human psyche that colors how we see consciousness.”
“For me, this means that we need to be really careful before we assume that there is any real mystery going on.”
The evolutionary roots of dualism
Berent blames what she calls “delusional attitudes about bodies and minds” to “the same old psychological biases that I’ve been studying in my lab for years.”
She calls the separation of mind and body dualism Previous research by Berent shows that autistic people are less dualistic than neurotypical people and that males are less dualistic than females .
Evolution is responsible for the fact people hold two different systems of perception in their mind, Berent says.
“Animals have an evolutionary advantage to be able to perceive objects, say, the bodies of their mothers,” she says.
It’s also important to be able to perceive objects that have agency as separate from other objects, Berent says. “You want to follow the mother and not a body that is inanimate” to receive nurturance and protection.
This type of dualism, she says, “primes us to think about people and their minds and bodies as separate from each other. That’s one reason we think about consciousness as this ethereal thing separate from the body.”
“The point is that our perception of consciousness changes depending on the situation. And if that’s the case, there’s no way that we can trust it to reflect what our consciousness really is. It must be that our brain plays tricks on us.”
An intimate understanding of who we are
“Every psychology student that has ever come into my class asks if we’re going to talk about consciousness,” Berent says.
“This is considered to be super important. This is our intimate understanding of who we are.”
Berent says the thought experiment outlined in her paper provides “the smoking gun” that intuitions about consciousness existing outside the body are manufactured by humans’ dualistically inclined brains.
Consciousness likely comes down to electrochemical functions in the brain, she says. “It’s hard for psychological reasons.”
Science & Technology
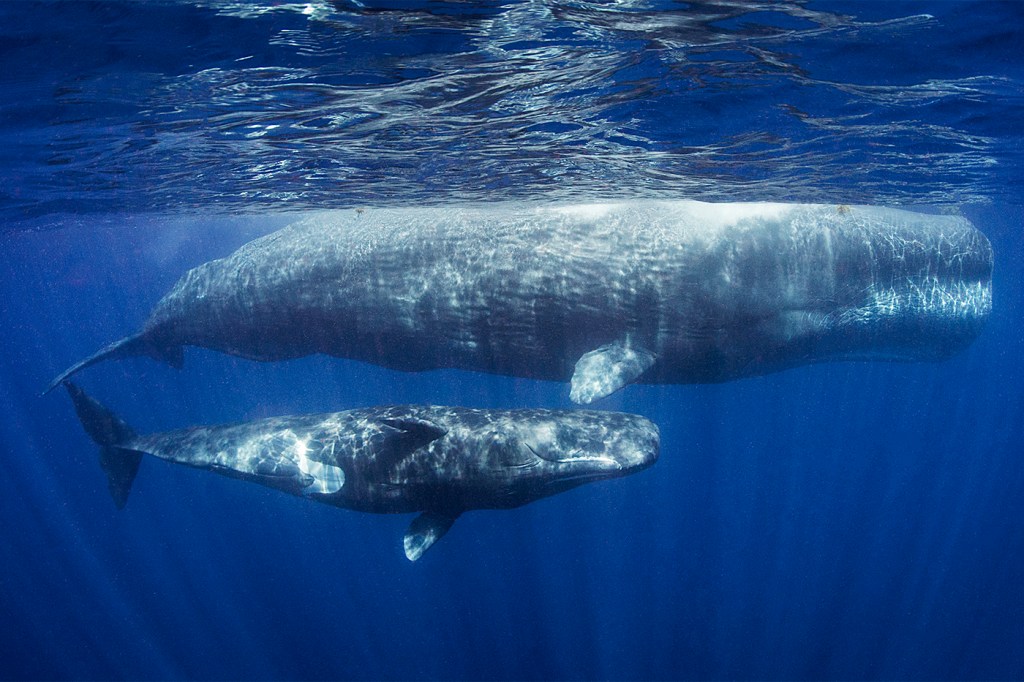
Recent Stories
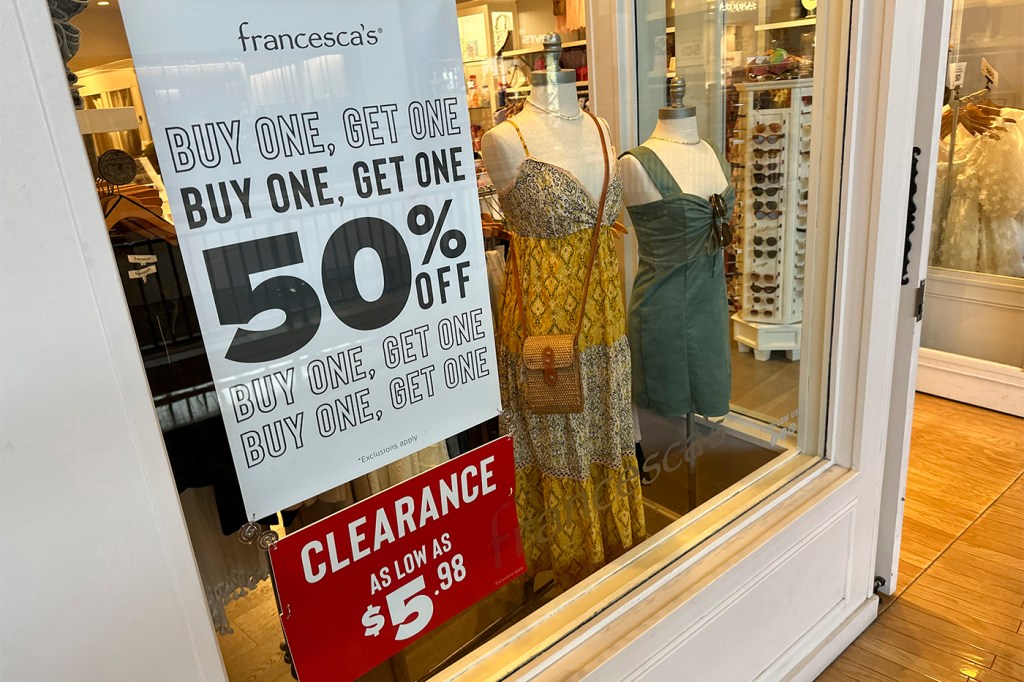
Thank you for visiting nature.com. You are using a browser version with limited support for CSS. To obtain the best experience, we recommend you use a more up to date browser (or turn off compatibility mode in Internet Explorer). In the meantime, to ensure continued support, we are displaying the site without styles and JavaScript.
- View all journals
Cognitive neuroscience articles from across Nature Portfolio
Cognitive neuroscience is the field of study focusing on the neural substrates of mental processes. It is at the intersection of psychology and neuroscience, but also overlaps with physiological psychology, cognitive psychology and neuropsychology. It combines the theories of cognitive psychology and computational modelling with experimental data about the brain.
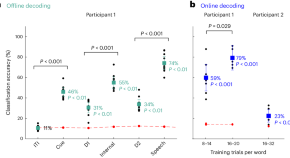
Brain–machine-interface device translates internal speech into text
For patients affected by speech disorders, brain–machine-interface (BMI) devices could restore their ability to verbally communicate. In this work, we captured neural activity associated with internal speech — words said within the mind with no associated movement or audio output — and translated these cortical signals into text in real time.
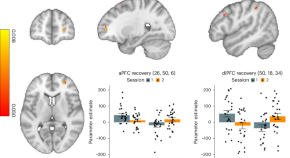
Prefrontal cortex activity increases after inpatient treatment for heroin addiction
Using task-based functional MRI, we examined inpatients with heroin use disorder. We found that 15 weeks of medication-assisted treatment (including supplemental group therapy) improved impaired anterior and dorsolateral prefrontal cortex function during an inhibitory control task. Inhibitory control, a core deficit in drug addiction, may be amenable to targeted prefrontal cortex interventions.
Related Subjects
- Cognitive control
- Consciousness
- Intelligence
- Personality
- Problem solving
Latest Research and Reviews
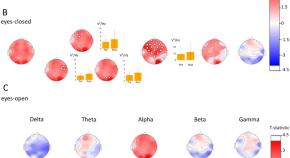
Short-term meditation training alters brain activity and sympathetic responses at rest, but not during meditation
- Anna Rusinova
- Maria Volodina
- Alexei Ossadtchi
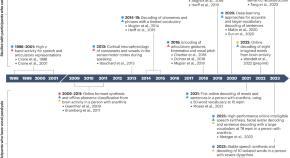
The speech neuroprosthesis
A clinically viable speech neuroprosthesis could restore natural speech to individuals with vocal-tract paralysis. In this Review, Silva et al. discuss rapid progress in neural interfaces and computational algorithms for decoding speech from cortical activity and propose evaluation metrics to help standardize speech neuroprostheses.
- Alexander B. Silva
- Kaylo T. Littlejohn
- Edward F. Chang
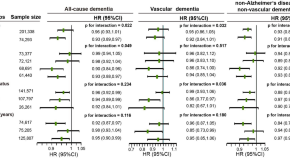
Association between household size and risk of incident dementia in the UK Biobank study
- Chao-Hua Cong
- Pan-Long Li
- Jing-Jing Su
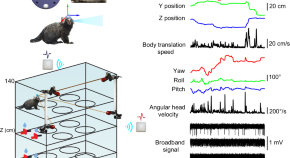
Primacy of vision shapes behavioral strategies and neural substrates of spatial navigation in marmoset hippocampus
How diurnal primates develop exploration-navigation strategy and how the physiology of primate hippocampus is shaped in navigation are not fully understood. Here authors show that marmosets adapted their navigation strategies to their diurnal ecological niche. Notably, marmoset hippocampal neurons are specialized for encoding combinations of view, head direction and place, and that theta oscillations are triggered by rapid head-gaze movements.
- Diego B. Piza
- Benjamin W. Corrigan
- Julio Martinez-Trujillo
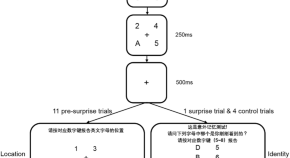
Children exhibit superior memory for attended but outdated information compared to adults
Children typically exhibit weaker memory than adults. Here, the authors report a developmental reversal-like phenomenon that children show better memory for attended but outdated information, suggesting underdeveloped memory selection in children.
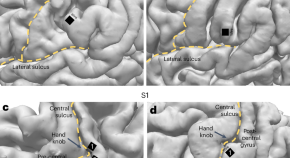
Representation of internal speech by single neurons in human supramarginal gyrus
Wandelt et al. describe a brain–machine interface that captures intracortical neural activity during internal speech (words said within the mind with no associated movement or audio output) and translates those cortical signals into real-time text.
- Sarah K. Wandelt
- David A. Bjånes
- Richard A. Andersen
News and Comment
Situational models of implicit bias.
- Maximilian A. Primbs
Mapping the claustrum to elucidate consciousness
- Navona Calarco
Prenatal alcohol exposure influences visual processing in infants
- Teresa Schubert
Attentional capture
A large network of brain regions is involved in salient distractor processing.
- Isobel Leake
Quick links
- Explore articles by subject
- Guide to authors
- Editorial policies

- International edition
- Australia edition
- Europe edition
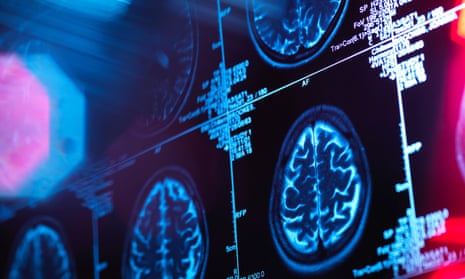
Sleep does not help brain wash out toxins, study suggests
Finding that clearance of fluid in mice brains is lower in sleep and anaesthesia runs counter to dominant view in neuroscience
The restorative effect of a good night’s rest is widely recognised and the popular scientific explanation has been that the brain washes out toxins during sleep.
However, new findings suggest this theory, which has become a dominant view in neuroscience, could be wrong. The study found that the clearance and movement of fluid in the brains of mice was, in fact, markedly reduced during sleep and anaesthesia.
“It sounded like a Nobel prize-winning idea,” said Prof Nick Franks, a professor of biophysics and anaesthetics at Imperial College London, and co-lead of the study.
“If you are sleep-deprived, countless things go wrong – you don’t remember things clearly, hand-eye coordination is poor,” he added. “The idea that your brain is doing this basic housekeeping during sleep just seems to make sense.”
However, there was only indirect evidence that the brain’s waste-removal system ramps up activity during sleep, Franks said.
In the latest study, published in the journal Nature Neuroscience , researchers used a fluorescent dye to study the brains of mice. This allowed them to see how quickly the dye moved from fluid-filled cavities, called the ventricles, to other brain regions and enabled them to measure the rate of clearance of the dye from the brain directly.
The study showed that the clearance of the dye was reduced by about 30% in sleeping mice, and 50% in mice that were under anaesthetic, compared with mice that were kept awake.
“The field has been so focused on the clearance idea as one of the key reasons why we sleep, and we were of course very surprised to observe the opposite in our results,” said Franks. “We found that the rate of clearance of dye from the brain was significantly reduced in animals that were asleep, or under anaesthetic.”
The researchers predict that the findings will extend to humans as sleep is a core need shared by all mammals.
Prof Bill Wisden, the interim director of the UK Dementia Research Institute at Imperial College London and co-lead author, said: “There are many theories as to why we sleep, and although we have shown that clearing toxins may not be a key reason, it cannot be disputed that sleep is important.”
The findings have relevance for dementia research due to the increasing evidence of a link between poor sleep and Alzheimer’s risk. It has not been clear whether lack of sleep might cause Alzheimer’s, or whether it is simply an early symptom. Some had hypothesised that without enough sleep, the brain may not be able to clear toxins effectively, but the latest research raises doubts about the plausibility of this explanation.
“Because that idea has held such sway, it’s probably increased people’s anxiety that if they don’t sleep they’ll be more likely to develop dementia,” said Franks.
Wisden said: “Disrupted sleep is a common symptom experienced by people living with dementia. However, we still do not know if this is a consequence or a driving factor in the disease progression. It may well be that having good sleep does help to reduce dementia risk for reasons other than clearing toxins.”
He added: “The other side to our study is that we have shown that brain clearance is highly efficient during the waking state. In general, being awake, active and exercising may more efficiently clean the brain of toxins.”
- Neuroscience
- Medical research
Most viewed
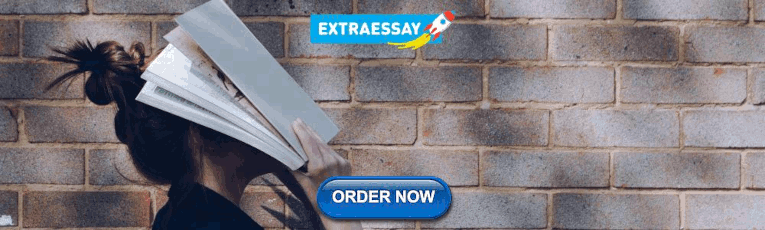
IMAGES
VIDEO
COMMENTS
Neuroscience research: 6 fascinating findings By Tim Newman on March 19, 2020 — Fact checked by Carolyn Robertson In this feature, we discuss six studies that uncover new and unexpected truths ...
Neuroscience is a multidisciplinary science that is concerned with the study of the structure and function of the nervous system. It encompasses the evolution, development, cellular and molecular ...
Brain clearance is reduced during sleep and anesthesia. It has been widely believed that a key function of sleep is to actively clear metabolites and toxins from the brain. Miao, Luo et al. show ...
New Study Reveals Age-Related Brain Changes Influence Recovery After Stroke. May 6, 2024 — A new study has revealed that areas of age-related damage in the brain relate to motor outcomes after a ...
Most neuroscience studies have focused on research related to cognitive functions, such as attention, memory and decision-making. In addition to these cognitive studies, there is also the implicit nature of mindsets that lead to the malleability of self-attributes (e.g., intelligence) . Subtle feedback and messages related to growth mindset can ...
Nature Neuroscience is a Transformative Journal; ... revealing connections between previously unrelated results from studies of case-control neuroimaging, differential gene expression, and ...
Emotion just wasn't accepted as an integral, intrinsic part of behavioral neuroscience, her field of study. That didn't make any sense to Tye. She decided to go her own way to become a leading ...
Neuroscience News Home. Neuroscience News is an independent open access science magazine. Since 2001, we have featured neuroscience research news from labs, universities, hospitals and news departments around the world. Topics include brain research, AI, psychology, neuroscience, mental health and neurotech.
Macaque study illuminates how brain perceives static images amid constant eye movements Read more. Reflections on Neurobiology, Past and Present ... of the interdisciplinary approach established by the Department's founders in 1966 continues today in our nearly 30 research laboratories that study neuroscience at the molecular, cellular ...
Here, we take a look back over some of the most popular neuroscience research articles of the year. Source: Neuroscience News. For over 20 years, Neuroscience News has reported on the latest, ground-breaking neuroscience research. ... A new meta-analysis of 15 studies reveals the optimum number of steps people of different age ranges should ...
Electrophysiological correlates of dentate nucleus deep brain stimulation for post-stroke motor recovery
The resources listed below are for gene expression-related information relevant to neuroscience research. Strategic Plan NINDS supports and performs a broad array of rigorous and important neuroscience research from fundamental studies of basic nervous system function to studies to improve treatments and prevent neurological disorders.
Neuroscience Research is an international journal for high quality articles in all branches of neuroscience, from the molecular to the behavioral levels. The journal is published in collaboration with the Japan Neuroscience Society and is open to all contributors in the world. Neuroscience Research publishes original full-length research ...
This simple fact, combined with the relatively inexpensive price (∼$50), make Guide to Research Techniques in Neuroscience an invaluable tool for students and instructors alike. With the continual emergence of new and modified techniques to study the nervous system, I hope that there are many future editions of the Guide in years to come.
Behavioral neuroscience, also called biological psychology, is the study of how the brain and the rest of the nervous system provide the foundation for behavior. It examines the neural basis of ...
The Brain Basis of Mental Illness. In 1990, U.S. President George H. W. Bush signed a proclamation designating 1990- 2000 as the "Decade of the Brain" to promote considerable neuroscience research efforts and raise public awareness of neurobiologically based conditions, such as schizophrenia and Alzheimer's disease (Goldstein, 1990).After nearly three decades, incredible progress has ...
The new study, published in the journal Nature Medicine, analyzed data from over 500 people with two copies of APOE4, a significantly larger pool than in previous studies. The researchers found ...
Neuroscience, the study of the nervous system, is a field that investigates the biological mechanisms that underlie behavior and how brains process information. The study of neuroscience provides both a broad scientific training and a deep understanding of the biology of the nervous system. Given the diversity of interests in this field, the ...
The approach the majority of neuroscientists take to the question of how consciousness is generated, it is probably fair to say, is to ignore it. Although there are active research programs looking at correlates of consciousness, and explorations of informational properties of what might be relevant neural ensembles, the tacitly implied mechanism of consciousness in these approaches is that it ...
The research, published in the journal Nature and co-led by Rockefeller University, sought to unravel how the brain's ability to retain and process information, known as working memory, improves ...
Top 100 in Neuroscience. This collection highlights our most downloaded* neuroscience papers published in 2021. Featuring authors from around the world, these papers showcase valuable research ...
This book reports on theoretical and experimental research answering key questions in neuroscience, philosophy of mind, and cognitive research. It gives a special emphasis on findings achieved within the territory of the former U.S.S.R, which has remained largely unknown to an international readership. The volume gathers authoritative studies ...
Widely used in neuroscience research, various experimental (animal) models may help improve our understanding of how different colors impact brain and behavioral processes. Complementing laboratory rodents, the zebrafish (Danio rerio) is rapidly emerging as an important novel model species to explore complex neurobehavioral processes.
Neuroscience is the study of how the nervous system develops, its structure, and what it does. The nervous system affects all parts of the human body, and neuroscientists may work in neurochemisty ...
Modern neuroscience is making significant progress in the study of brain functions, which can be of great importance for the education. However, there is a gap between current neuroscientific evidence on how the brain learns and its direct application in classrooms. What can neuroscientists, psychologists, and educators do to improve the interaction between neuroscience and education?
The study involved 21 young adult participants, all of whom were non-musicians to avoid the confounding effects of musical training on brain activity patterns. These participants were exposed to ...
Northeastern research says. In the Neuroscience of Consciousness, psychology professor Iris Berent argues that the debate stems from the delusional biases in the way humans think about the separation, or lack thereof, between body and mind. by Cynthia McCormick Hibbert May 10, 2024 Erroneous but natural human perceptions are the reason the ...
Nature Neuroscience presents a special focus issue that highlights advances in methods, analyses and practices across scales of investigation and subfields of neuroscience. Single-cell ...
RSS Feed. Cognitive neuroscience is the field of study focusing on the neural substrates of mental processes. It is at the intersection of psychology and neuroscience, but also overlaps with ...
In the latest study, published in the journal Nature Neuroscience, researchers used a fluorescent dye to study the brains of mice. This allowed them to see how quickly the dye moved from fluid ...