Sorry. You need a frames capable broswer to view this page.
- Search Menu
- Cytogenetics
- Cytotechnology
- Histotechnology
- Management/Administration
- Microbiology
- Molecular Pathology
- Molecular Biology
- Transfusion Medicine
- Advance articles
- COVID-19 articles
- Current Virtual Issue
- Cover Archive
- Author Guidelines
- Open Access
- Submission Site
- Why publish?
- Advertising and Corporate Services
- Advertising
- Reprints and ePrints
- Sponsored Supplements
- Branded Books
- About Laboratory Medicine
- About the American Society for Clinical Pathology
- Editorial Board
- Self-Archiving Policy
- Journals on Oxford Academic
- Books on Oxford Academic
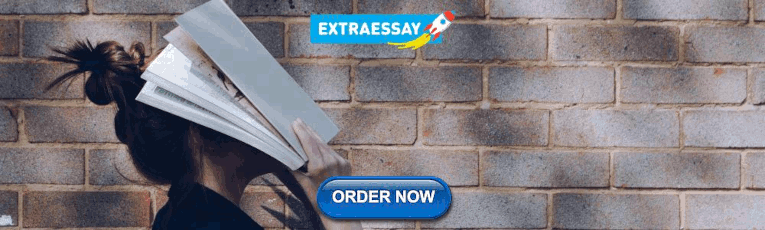
Article Contents
- < Previous
Renal Function Tests: A Clinical Laboratory Perspective
- Article contents
- Figures & tables
- Supplementary Data
Henry O. Ogedegbe, Renal Function Tests: A Clinical Laboratory Perspective, Laboratory Medicine , Volume 38, Issue 5, May 2007, Pages 295–304, https://doi.org/10.1309/RWG5DY7RG1CYBUR7
- Permissions Icon Permissions
Worldwide, chronic kidney disease has become a public health problem with increasing prevalence and high cost for treatment and prevention. It is projected that by 2030 there will be about 2 million patients needing dialysis and transplantation. Kidneys perform vital functions in the homeostatic processes of the human body including the filtration of blood, maintenance of acid base, water and electrolyte balance, regulation of hormone levels, and removal of waste products and unwanted substances from plasma. Various hormones that have metabolic and physiological functions such as erythropoietin, the prostaglandins, and so forth, are produced by the kidneys. Several diseases, such as acute nephrotic syndrome, acute glomerulonephritis, and others, can singly or in combination precipitate renal diseases. Various clinical laboratory tests are useful in the investigation and evaluation of kidney function. Different treatment options available for the management and prevention of renal disease complications include dialysis and transplantation.
- nephrotic syndrome
- prostaglandins
- hemodialysis
- kidney diseases
- glomerulonephritis
- renal function
- kidney failure, chronic
- electrolytes
- erythropoietin
- kidney function tests
- laboratory techniques and procedures
- waste products
- dialysis procedure
- public health medicine
- transplantation
Email alerts
More on this topic, related articles in pubmed, citing articles via.
- Recommend to your Library
Affiliations
- Online ISSN 1943-7730
- Print ISSN 0007-5027
- Copyright © 2024 American Society for Clinical Pathology
- About Oxford Academic
- Publish journals with us
- University press partners
- What we publish
- New features
- Open access
- Institutional account management
- Rights and permissions
- Get help with access
- Accessibility
- Media enquiries
- Oxford University Press
- Oxford Languages
- University of Oxford
Oxford University Press is a department of the University of Oxford. It furthers the University's objective of excellence in research, scholarship, and education by publishing worldwide
- Copyright © 2024 Oxford University Press
- Cookie settings
- Cookie policy
- Privacy policy
- Legal notice
This Feature Is Available To Subscribers Only
Sign In or Create an Account
This PDF is available to Subscribers Only
For full access to this pdf, sign in to an existing account, or purchase an annual subscription.

Introduction
Case report, statement of ethics, disclosure statement, funding sources, author contributions, a case of acute kidney injury in a patient with renal hypouricemia without intense exercise.
- Split-Screen
- Article contents
- Figures & tables
- Supplementary Data
- Peer Review
- Open the PDF for in another window
- Get Permissions
- Cite Icon Cite
- Search Site
Daiki Aomura , Kosuke Sonoda , Makoto Harada , Koji Hashimoto , Yuji Kamijo; A Case of Acute Kidney Injury in a Patient with Renal Hypouricemia without Intense Exercise. Case Rep Nephrol Dial 12 May 2020; 10 (1): 26–34. https://doi.org/10.1159/000506673
Download citation file:
- Ris (Zotero)
- Reference Manager
Exercise-induced acute kidney injury (EIAKI) frequently develops in patients with renal hypouricemia (RHUC). However, several cases of RHUC with acute kidney injury (AKI) but without intense exercise have been reported. We encountered a 15-year-old male with RHUC who experienced AKI. He reported no episodes of intense exercise and displayed no other representative risk factors of EIAKI, although a vasopressor had been administered for orthostatic dysregulation before AKI onset. His kidney dysfunction improved with discontinuation of the vasopressor and conservative treatment. Thus, AKI can develop in patients with RHUC in the absence of intense exercise, for which vasopressors may be a risk factor.
Exercise-induced acute kidney injury (EIAKI) is a major complication in patients with renal hypouricemia (RHUC). EIAKI usually develops after intense exercise, such as anaerobic exertion, and is not accompanied by rhabdomyolysis [ 1 ]. However, there are several case reports of patients experiencing EIAKI without intense exercise [ 2-4 ]. Although the pathomechanism and risk factors of EIAKI remain unclear, many reports suggest that an oxidation-reduction imbalance is associated with EIAKI onset [ 5 ]. We herein report a case of acute kidney injury (AKI) in a patient with RHUC in the absence of intense exercise, which may have been caused by an oral vasopressor.
A 15-year-old male complained of strong fatigue after intense exercise since childhood. He had no remarkable medical history apart from allergic rhinitis. After entering high school, he often felt unwell, especially in the morning, and frequently missed classes. He was diagnosed as having orthostatic dysregulation and prescribed amezinium metilsulfate 10 mg/day, but his symptoms persisted. Eight days after the start of treatment he was switched to etilefrine 5 mg/day. However, his fatigue progressively worsened. He was found vomiting and unresponsive after collapsing in the bathroom on the eighth night following the prescription change and taken to the hospital by his family. In the emergency room he exhibited mild consciousness disturbance (Glasgow Coma Scale: E4V4M6) and complained of right lower abdominal pain. Laboratory tests (blood and urine), whole-body computed tomography, and head magnetic resonance imaging did not indicate any abnormalities (serum creatinine level 1.0 mg/dL, uric acid level 7.2 mg/dL). His conscious state and abdominal pain were improved on the next day, but his blood pressure gradually increased from 100/60 to 180/80 mm Hg and his serum creatinine level rose from 1.0 to 5.5 mg/dL during 5 days of admission. He was then transferred to our institution for the treatment of AKI and severe hypertension.
At the time of admission to our hospital the patient was fully conscious and alert. His body temperature was 37.2°C, blood pressure was 161/98 mm Hg, heart rate was 83 beats/min, and respiratory rate was 17 breaths/min. His height was 174 cm and his body weight was 54 kg. Physical examination detected no signs of dehydration, rash, or other abnormalities of the neck, chest, abdomen, or extremities. He had been taking loratadine 10 mg/day for his allergic rhinitis for several months. Both loratadine and etilefrine had been discontinued upon admission to the previous hospital. There was no family history of kidney dysfunction, and he reported no episodes of intense exercise other than daily commuting by bicycle to school. No alcohol consumption, smoking, or illegal drug use were reported. His laboratory data at the time of transfer to our hospital are summarized in Table 1 . Urinalysis showed mild proteinuria (0.66 g/gCr) and elevation of the tubulointerstitial injury marker β2 microglobulin (1,498 μg/L). Hematuria was not observed. His serum level of uric acid was low at 3.2 mg/dL and his fractional excretion of uric acid was high at 49.7%. Laboratory markers of rhabdomyolysis, diabetes mellitus, infection, and collagen diseases such as creatine phosphokinase, hemoglobin A1c, C-reactive protein, and autoimmune antibodies were all within normal range. An electrocardiogram disclosed left anterior hemiblock and nonspecific intraventricular conduction delay that had been detected when he was an elementary school student. A chest X-ray revealed no abnormalities. Ultrasound echography showed bilateral mild kidney swelling with increased renal cortical echogenicity (Fig. 1 ). No stenotic lesions were detected in the aorta or renal arteries, although the resistance index of the intrarenal arteries was slightly high (left 0.69, right 0.69), indicating a circulatory disturbance in the renal microvessels. Hydronephrosis and renal calcification were absent. An ultrasound-guided kidney biopsy performed 3 days after arrival at our hospital showed mild interstitial edema, vascular endothelial cell swelling in the renal interlobular arterioles, and no obvious signs of acute tubular necrosis (ATN) (Fig. 2 ). Treatment with continuous intravenous infusion of extracellular fluids and nicardipine gradually improved his kidney function and hypertension. His serum uric acid level decreased to 1.0 mg/dL (Fig. 3 ), and his fractional excretion of uric acid was at 55.9% at 10 days after admission. He was ultimately diagnosed as having AKI with RHUC and discharged 12 days after transfer to our hospital. Hypouricemia was found in his parents and a sister, indicating a hereditary condition. However, genetic screening did not detect any known causative RHUC mutations on URAT1/SLC22A12 or GLUT9/SLC2A9 .
Main laboratory data on admission to our hospital
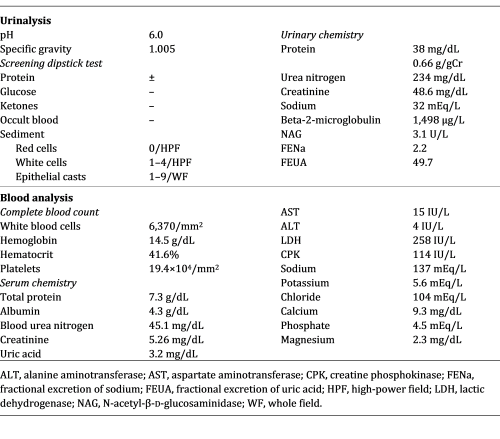
Renal ultrasound showed mild kidney swelling with increased renal cortical echogenicity. Hydronephrosis and renal calcification were not observed. Renal imaging findings were similar bilaterally (left 105 × 62 mm, right 115 × 63 mm).
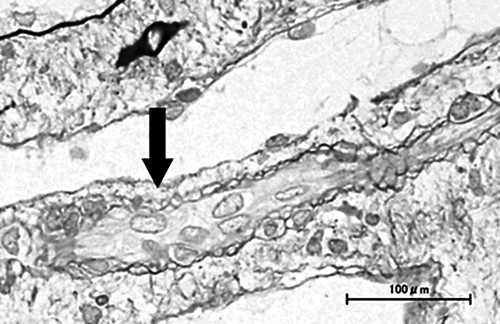
Kidney biopsy specimen findings. Mild interstitial edema and vascular lumen narrowing by endothelial cell swelling (arrow) were detected (periodic acid-methenamine silver stain). No other abnormalities were found, including signs of acute tubular necrosis.
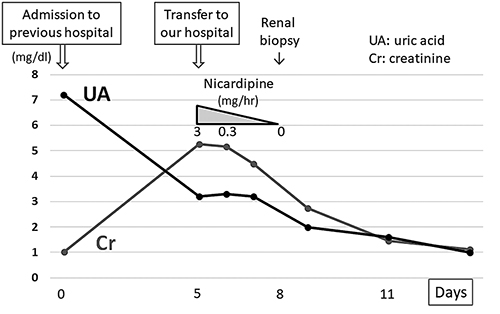
Clinical course of the present case. Vasopressors that had been administered for 15 days were discontinued on admission. After transfer to our hospital, his renal function improved gradually with continuous intravenous infusion of extracellular fluids and nicardipine. The serum uric acid level decreased steadily to 1.0 mg/dL during hospitalization.
Ishikawa et al. [ 6 ] first described EIAKI as AKI with accompanying abdominal or lower back pain after intense exercise, such as a 100-meter dash. EIAKI is differentiated from AKI with rhabdomyolysis by normal or slightly elevated serum myoglobin and creatine phosphokinase levels. EIAKI typically occurs in young males, with more than half having RHUC. Enhanced computed tomography often displays a wedge-shaped contrast defect in the kidneys. As for the clinical course of EIAKI, kidney dysfunction improves naturally without any special treatment [ 1, 7 ]. Although the reported patient had no intense episodes of exercise, EIAKI was diagnosed because he had RHUC, his kidney function recovered naturally, and he was young and male.
Blood pressure and serum creatinine level in our patient increased gradually following admission to the former hospital. As high blood pressure alone might cause AKI, we could not exclude the possible involvement of hypertension in AKI development. However, his serum creatinine level ultimately improved to 0.7 mg/dL after the final discharge despite having been 1.0 mg/dL on first admission, indicating that it had already been elevated by 0.3 mg/dL at the former hospital. Considering the fact that his blood pressure was normal on admission, AKI was thought to have developed before blood pressure elevation. Furthermore, his serum uric acid level was much higher on first admission (7.2 mg/dL) than at discharge (1.0 mg/dL), suggesting AKI onset prior to the former hospital visit. We suspected that AKI caused hypertension, which in turn worsened AKI. The elevation of blood pressure was assumed to be an exacerbation factor of EIAKI rather than its main cause.
The reported patient had no intense episodes of exercise. Lee et al. [ 3 ] described 17 AKI patients with abdominal or lower back pain who exhibited the characteristic patchy kidney sign on enhanced computed tomography. Among them, 5 patients reported no episodes of intense exercise. To the best of our knowledge, there have been 8 patients with EIAKI who did not have any episodes of intense exertion [ 2-4 ], with 5 experiencing infection or analgesic usage before EIAKI onset (Table 2 ), thought to be risk factors of EIAKI in addition to RHUC [ 3, 8, 9 ]. These reports support the notion that EIAKI can develop without intense exercise and the existence of risk factors other than strong exertion. However, to date no reports have focused on the relationship between lack of intense exercise and the etiology and development mechanism of EIAKI.
Clinical findings of current and previous reported cases of EIAKI without strenuous exercise
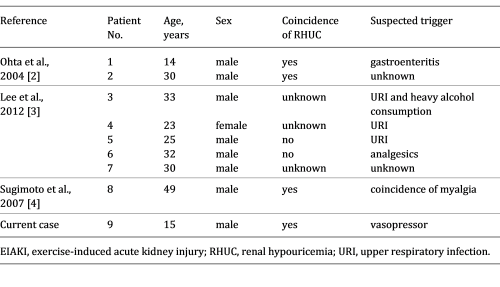
The pathomechanism of EIAKI is unclear, but renal circulatory disturbance by reactive oxygen species (ROS) is thought to be a main cause [ 5 ]. Intense exercise, such as anaerobic exertion, produces large amounts of ROS, which are rapidly removed by uric acid and other scavengers in the healthy population [ 8 ]. Patients with RHUC have insufficient scavengers, resulting in inadequate ROS removal and the subsequent activation of vasoconstrictive factors, vasoconstriction, and renal ischemia [ 2 ]. Since renal vasoconstriction is known to trigger further vasoconstriction and oxidative stress via activation of the renin-angiotensin system and blood pressure elevation [ 10 ], EIAKI patients are thought to show a vicious cycle between oxidative stress and vasoconstriction – oxidative stress causes stronger vasoconstriction and vasoconstriction causes more oxidative stress – culminating in acute and severe renal injury. In the present case, the patient had been taking vasopressors for orthostatic dysregulation for 15 days prior to the onset of AKI. Amezinium metilsulfate inhibits monoamine oxidase activity and suppresses the uptake of noradrenaline, while etilefrine activates type α1 and β1 adrenaline receptors. Thus, both vasopressors increased cardiac output and the constriction of peripheral vessels [ 11, 12 ]. Bellomo et al. [ 13 ] reported that activation of type α1 adrenaline receptors could cause excessive renal vasoconstriction and decreased renal blood flow in models of healthy renal hemodynamics. Radaković et al. [ 14 ] described that adrenaline induction increased ROS and caused a disruption in oxidant/antioxidant balance. Considering these results and the developmental mechanism of EIAKI (i.e., ROS and renal ischemia), we suspect that the vasopressors may have affected the onset or worsening of EIAKI by increasing ROS, exacerbating vasoconstriction, and forming a vicious cycle of diminished renal hemodynamics. Karasawa et al. [ 15 ] reported a case of EIAKI who was given midodrine, another vasopressor, before the onset of EIAKI, and Saito et al. [ 16 ] described that vasoexpansion by low-dose dopamine improved the resistance index of renal arterioles in 2 cases of EIAKI, implying the relation between vasopressors and EIAKI in clinical settings. Although no studies have directly addressed the relationship between vasopressors and EIAKI, past reports and our own results indicate an importance of catecholamine level homeostasis in the pathogenesis of EIAKI. We suspect that vasopressors may be associated with AKI onset in RHUC patients and may be a risk factor of EIAKI.
Renal biopsy showed no significant abnormalities in the present case. Although patients with EIAKI generally exhibit ATN, Ohta et al. [ 2 ] reported no abnormalities in 6 of 28 renal biopsies from EIAKI patients, which implied that EIAKI could develop without ATN. AKI with renal ischemia often causes ATN. However, tubular necrosis is sometimes absent without a sufficient degree or duration of ischemia, and early treatment for renal ischemia leads to a rapid improvement in renal function in such cases [ 17 ]. In the present patient, vasopressors, which might be a risk factor for EIAKI, were discontinued and intravenous antihypertensive medication was induced just after the first admission. The serum uric acid level was temporarily elevated on admission by AKI, and the patient’s scavenging ability with serum uric acid was thought to be temporarily improved. These factors could have mitigated the vicious cycle between renal vasoconstriction and oxidative stress, reduced the severity of renal ischemia, and prevented ATN development. However, as no studies have addressed the cause or meaning of a lack of ATN in some EIAKI patients, a greater number of studies are needed.
In conclusion, AKI can develop in patients with RHUC without intense exercise, possibly through the use of vasopressors. Further related case reports are needed to clarify the association between vasopressor use and AKI in patients with RHUC.
The present case report adhered to the Declaration of Helsinki. Informed consent for publication was obtained from the patient.
The authors declare no conflicts of interest.
The authors received no specific funding for this work.
D. Aomura drafted the article. K. Sonoda, M. Harada, and K. Hashimoto revised the article critically for important intellectual content. Y. Kamijo revised the article critically for important intellectual content and gave final approval of the version to be submitted.

Email alerts
Citing articles via, suggested reading.
- Online ISSN 2296-9705
INFORMATION
- Contact & Support
- Information & Downloads
- Rights & Permissions
- Terms & Conditions
- Catalogue & Pricing
- Policies & Information
- People & Organization
- Stay Up-to-Date
- Regional Offices
- Community Voice
SERVICES FOR
- Researchers
- Healthcare Professionals
- Patients & Supporters
- Health Sciences Industry
- Medical Societies
- Agents & Booksellers
Karger International
- S. Karger AG
- P.O Box, CH-4009 Basel (Switzerland)
- Allschwilerstrasse 10, CH-4055 Basel
- Tel: +41 61 306 11 11
- Fax: +41 61 306 12 34
- Contact: Front Office
- Experience Blog
- Privacy Policy
- Terms of Use
This Feature Is Available To Subscribers Only
Sign In or Create an Account
Acute Renal Failure
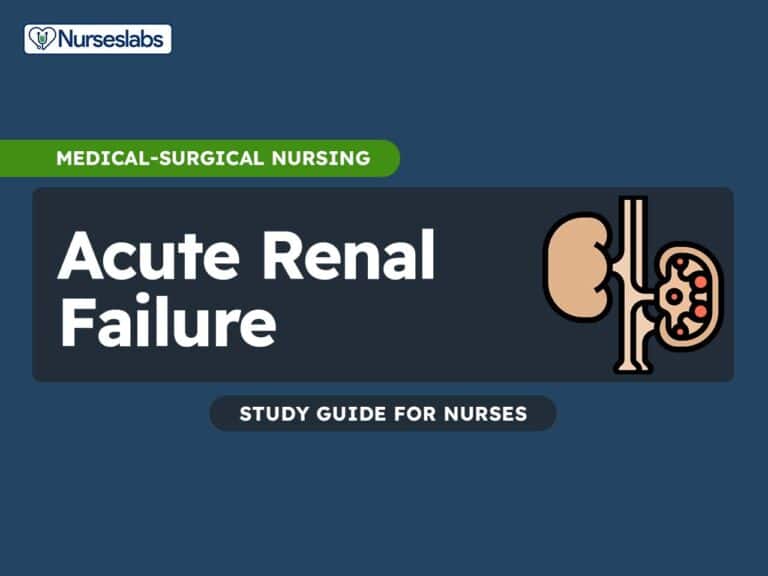
Learn about the nursing care management of patients with acute renal failure in this nursing study guide .
Table of Contents
- What is Acute Renal Failure?
Pathophysiology
Statistics and incidences, clinical manifestations, complications, urine tests, blood tests, other tests, medical management, nursing assessment, nursing diagnosis, nursing care planning & goals, nursing interventions, discharge and home care guidelines, documentation guidelines, what is acute renal failure.
Renal failure results when the kidneys cannot remove the body’s metabolic wastes or perform their regulatory functions.
- Acute renal failure (ARF) is a rapid loss of renal function due to damage to the kidneys.
- Acute renal failure is also known today as acute kidney injury (AKI) .
- It is a problem seen in hospitalized patients and those in outpatient settings.
- A healthy adult eating a normal diet needs a minimum daily urine output of approximately 400 ml to excrete the body’s waste products through the kidneys. An amount lower than this indicates a decreased GFR.
Although the pathogenesis of ARF and oliguria is not always known, many times there is a specific underlying problem.
- Underlying problems. There are underlying problems that cause the development of ARF such as hypovolemia , hypotension , reduced cardiac output and failure, and obstruction of the kidney.
- Blood flow. As these underlying problems affect the body, the blood flow to the kidneys reduces.
- Decreased kidney function. With inadequate blood flow to the kidney, there is impaired kidney function.
- Failure. If the underlying conditions are not treated and corrected, they can lead to permanent damage of the kidneys.
Here’s the statistics and incidences for acute renal failure:
- ARF affects approximately 1% of patients on admission to the hospital, 2% to 5% during the hospital stay, 4% to 15% after cardiopulmonary bypass surgery , and 10% of cases acute renal failure occurs in isolation (i.e. single organ failure).
- In the United States, the annual incidence of acute renal failure is 100 cases for every million people . It’s diagnosed in 1% of hospital admissions. Hospital-acquired acute renal failure occurs in 4% of all admitted patients and 20% of patients who are admitted to critical care units.
Acute renal failure (ARF) has four well-defined stages: onset, oliguric or anuric, diuretic , and convalescent. Treatment depends on stage and severity of renal compromise. ARF can be divided into three major classifications, depending on site:
- Prerenal failure is caused by interference with renal perfusion (e.g., blood volume depletion, volume shifts [“third-space” sequestration of fluid], or excessive/too-rapid volume expansion), manifested by decreased glomerular filtration rate (GFR).
- Disorders that lead to prerenal failure include cardiogenic shock , heart failure (HF), myocardial infarction (MI), burns , trauma , hemorrhage , septic or anaphylactic shock , and renal artery obstruction.
Renal (or intrarenal)
- Intrarenal causes for renal failure are associated with parenchymal changes caused by ischemia or nephrotoxic substances.
- Acute tubular necrosis (ATN) accounts for 90% of cases of acute oliguria.
- Destruction of tubular epithelial cells results from (1) ischemia/hypoperfusion (similar to prerenal hypoperfusion except that correction of the causative factor may be followed by continued oliguria for up to 30 days) and/or (2) direct damage from nephrotoxins.
- Postrenal failure occurs as the result of an obstruction in the urinary tract anywhere from the tubules to the urethral meatus.
- Obstruction most commonly occurs with stones in the ureters, bladder , or urethra; however, trauma, edema associated with infection, prostate enlargement, and strictures also cause postrenal failure.
There are four phases of ARF: initiation, oliguria, diuresis, and recovery.
- Initiation. The initiation period begins with the initial insult, and ends when oliguria develops.
- Oliguria. The oliguria period is accompanied by an increase in the serum concentration of substances usually excreted by kidneys.
- Diuresis. The diuresis period is marked by a gradual increase in urine output, which signals that glomerular filtration has started to recover.
- Recovery. The recovery period signals the improvement of renal function and may take 3 to 12 months .
The causes of ARF depend on its categories: prerenal, intrarenal, and postrenal.
- Prerenal. Examples of prerenal causes are volume depletion, impaired cardiac efficiency, and vasodilation.
- Intrarenal. Examples of intrarenal causes are prolonged renal ischemia, nephrotoxic agents, and infectious processes.
- Postrenal. An example of a postrenal cause is urinary tract obstruction.
Almost every system of the body is affected by the failure of the normal renal regulatory mechanisms.
- Lethargy. Since waste products cannot be filtered, it slowly accumulates in the different parts of the body.
- Dryness. The skin and mucous membrane are dry from dehydration .
- Central nervous system symptoms. This include drowsiness, headache, muscle twitching, and seizures.
- Increased creatinine. All phases of ARF exhibit an increase in creatinine.
Preventing renal failure involves the following:
- Hydration. Provide adequate hydration to patients at risk for dehydration .
- Shock. Prevent and treat shock promptly with blood and fluid replacement.
- Close monitoring. Monitor central venous and arterial pressures and hourly urine output of critically ill patients to detect the onset of renal failure as early as possible.
- Blood administration. Take precautions to ensure that the appropriate blood is administered to the correct patient in order to avoid severe transfusion reactions.
- Infections. Prevent and treat infections promptly because they can produce progressive renal damage.
- Toxic drug effects. To prevent toxic drug effects, closely monitor dosage , duration of use, and blood levels of all medications metabolized or excreted by the kidneys.
Depending on the duration and severity of ARF, a wide range of potentially life-threatening complications can occur.
- Metabolic acidosis. Waste products could not be eliminated by the kidneys and they can contribute to metabolic acidosis.
- Fluid and electrolyte imbalances . Imbalances may occur due to hemorrhage , renal losses, and gastrointestinal losses.
Assessment and Diagnostic Findings
Assessment and diagnosis of a patient with ARF include evaluation for changes in the urine, diagnostic tests that evaluate the kidney contour, and a variety of normal laboratory values.
- Volume: Usually less than 100 mL/24 hr (anuric phase) or 400 mL/24 hr (oliguric phase), which occurs within 24–48 hr after renal insult. Nonoliguric (more than 400 mL/24 hr) renal failure also occurs when renal damage is associated with nephrotoxic agents (e.g., contrast media or antibiotics ).
- Color: Dirty, brown sediment indicates the presence of RBCs, hemoglobin, myoglobin, porphyrins.
- Specific gravity: Less than 1.020 reflects kidney disease, e.g., glomerulonephritis , pyelonephritis with loss of ability to concentrate; fixed at 1.010 reflects severe renal damage.
- pH: Greater than 7 found in urinary tract infections (UTIs), renal tubular necrosis, and chronic renal failure (CRF).
- Osmolality: Less than 350 mOsm/kg is indicative of tubular damage, and urine/serum ratio is often 1:1.
- Creatinine (Cr) clearance: Renal function may be significantly decreased before blood urea nitrogen (BUN) and serum Cr show significant elevation.
- Sodium: Usually increased if ATN is cause for ARF, more than 40 mEq/L if a kidney is not able to resorb sodium , although it may be decreased in other causes of prerenal failure.
- Fractional sodium (Fe Na ): Ratio of sodium excreted to total sodium filtered by the kidneys reveals the inability of tubules to reabsorb sodium. Readings of less than 1% indicate prerenal problems, higher than 1% reflect intrarenal disorders.
- Bicarbonate: Elevated if metabolic acidosis is present.
- Red blood cells (RBCs): May be present because of infection, stones, trauma, tumor , or altered glomerular filtration (GF).
- Protein: High-grade proteinuria (3–4+) strongly indicates glomerular damage when RBCs and casts are also present. Low-grade proteinuria (1–2+) and white blood cells (WBCs) may be indicative of infection or interstitial nephritis. In ATN, proteinuria is usually minimal.
- Casts: Usually signal renal disease or infection. Cellular casts with brownish pigments and numerous renal tubular epithelial cells are diagnostic of ATN. Red casts suggest acute glomerular nephritis.
- BUN/Cr: Elevated and usually rise in proportion with ratio of 10:1 or higher.
- Complete blood count (CBC): Hemoglobin (Hb) decreased in presence of anemia . RBCs often decreased because of increased fragility/decreased survival.
- Arterial blood gases ( ABGs ): Metabolic acidosis (pH less than 7.2) may develop because of decreased renal ability to excrete hydrogen and end products of metabolism. Bicarbonate decreased.
- Sodium: Usually increased, but may vary.
- Potassium: Elevated related to retention and cellular shifts (acidosis) or tissue release (red cell hemolysis).
- Chloride, phosphorus, and magnesium : Usually elevated.
- Calcium: Decreased.
- Serum osmolality: More than 285 mOsm/kg; often equal to urine.
- Protein: Decreased serum level may reflect protein loss via urine, fluid shifts, decreased intake, or decreased synthesis because of lack of essential amino acids.
- Radionuclide imaging: May reveal calicectasis, hydronephrosis, narrowing, and delayed filling or emptying as a cause of ARF.
- Kidney, ureter, bladder (KUB) x-ray: Demonstrates size of kidneys/ureters/bladder, presence of cysts, tumors, ad kidney displacement or obstruction (stones).
- Retrograde pyelogram: Outlines abnormalities of renal pelvis and ureters.
- Renal arteriogram: Assesses renal circulation and identifies extravascularities, masses.
- Voiding cystoureterogram: Shows bladder size, reflux into ureters, retention.
- Renal ultrasound: Determines kidney size and presence of masses, cysts, obstruction in upper urinary tract.
- Nonnuclear computed tomography (CT) scan: Cross-sectional view of kidney and urinary tract detects presence/extent of disease.
- Magnetic resonance imaging (MRI): Provides information about soft tissue damage.
- Excretory urography (intravenous urogram or pyelogram): Radiopaque contrast concentrates in urine and facilitates visualization of KUB.
- Endourology: Direct visualization may be done of urethra, bladder, ureters, and kidney to diagnose problems, biopsy, and remove small lesions and/or calculi.
- Electrocardiogram ( ECG ): May be abnormal, reflecting electrolyte and acid-base imbalances.
- Urinalysis: Analysis of the urine affords enormous insight into the function of the kidneys.
- Twenty–four–hour urine tests: This test requires you to collect all of your urine for 24 consecutive hours. The urine may be analyzed for protein and waste products (urea nitrogen and creatinine). The presence of protein in the urine indicates kidney damage. The amount of creatinine and urea excreted in the urine can be used to calculate the level of kidney function and the glomerular filtration rate (GFR).
- Glomerular filtration rate (GFR): The GFR is a standard means of expressing overall kidney function. As kidney disease progresses, GFR falls. The normal GFR is about 100–140 mL/min in men and 85–115 mL/min in women. It decreases in most people with age. The GFR may be calculated from the amount of waste products in the 24–hour urine or by using special markers administered intravenously. Patients are divided into five stages of chronic kidney disease based on their GFR.
- Urine Specific Gravity: This is a measure of how concentrated a urine sample is. A concentrated urine sample would have a specific gravity over 1.030 or 1.040
- Creatinine is a breakdown product of normal muscle breakdown.
- Urea is the waste product of breakdown of protein.
- The level of these substances rises in the blood as kidney function worsens.
- High potassium (hyperkalemia) is a particular concern.
- The acid–base balance of the blood is usually disrupted as well.
- Decreased production of the active form of vitamin D can cause low levels of calcium in the blood. Inability to excrete phosphorus by failing kidneys causes its levels in the blood to rise.
- Blood cell counts: Because kidney disease disrupts blood cell production and shortens the survival of red cells, the red blood cell count and hemoglobin may be low ( anemia ). Some patients may also have iron deficiency due to blood loss in their gastrointestinal system . Other nutritional deficiencies may also impair the production of red cells.
- In general, kidneys are shrunken in size in chronic kidney disease , although they may be normal or even large in size in cases caused by adult polycystic kidney disease, diabetic nephropathy, and amyloidosis.
- Biopsy: A sample of the kidney tissue (biopsy) is sometimes required in cases in which the cause of the kidney disease is unclear. Usually, a biopsy can be collected with local anesthesia only by introducing a needle through the skin into the kidney.
The objectives of treatment of ARF are to restore normal chemical balance and prevent complications until repair of renal tissue and restoration of renal function can occur.
- Pharmacologic therapy. Cation-exchange resins or Kayexalate can reduce elevated potassium levels; IV dextrose 50%, insulin , and calcium replacement may be administered to shift potassium back into cells; diuretic agents are often administered to control fluid volume.
- Nutritional therapy. Replacement of dietary proteins is individualized to provide the maximum benefit and minimize uremic symptoms; likewise, caloric requirements are met with high-carbohydrate meals, because carbohydrates have a protein-sparing effect; foods and fluids containing potassium or phosphorus are restricted; and after diuretic phase, the patient is placed on a high-protein , high-calorie diet.
Nursing Management
The nurse has an important role in caring for the patient with ARF.
Assessment usually focuses on the characteristics of the urine.
- Assess urine output. Urine output varies from scanty to a normal volume.
- Assess blood in the urine. Hematuria may be present in patients with ARF.
- Assess laboratory results. Laboratory results may increase, decrease, or stabilize and these may indicate each phase of ARF.
Based on the assessment data, appropriate nursing diagnoses for a patient with ARF include:
- Electrolyte imbalance related to increased potassium levels.
- Risk for deficient volume related to increased in urine output.
Main Article: 6 Acute Renal Failure Nursing Care Plans
The goals for a patient with ARF are:
- Improve nutritional intake.
- Restore fluid balance .
- Reduce metabolic rate.
- Promote pulmonary function.
- Prevent infection.
Nursing interventions are aimed at restoring renal function and reducing potential causes of increased renal injury.
- Monitor fluid and electrolyte balance. The nurse monitors the patient’s fluid and electrolyte levels and physical indicators of potential complications during all phases pf the disorder.
- Reducing metabolic rate. Bed rest is encouraged and fever and infection are prevented or treated promptly.
- Promoting pulmonary function. The patient is assisted to turn, cough , and take deep breaths frequently to prevent atelectasis and respiratory tract infection.
- Preventing infection. Asepsis is essential with invasive lines and catheters to minimize the risk of infection and increased metabolism.
- Providing skin care . Bathing the patient with cool water, frequent turning, and keeping the skin clean and well moisturized and keeping the fingernails trimmed to avoid excoriation are often comforting and prevent skin breakdown.
- Provide safety measures. Patient with CNS involvement may be dizzy or confused.
A successful nursing care plan has achieved the following:
- Improved nutritional intake.
- Restored fluid balance.
- Reduced metabolic rate.
- Promoted pulmonary function.
- Prevented infection.
The nurse plays an important role in teaching the patient and family with ARF.
- Nutrition . A referral to the nutritionist is made because of the dietary changes required.
- Problems to report. The patient and family must know what problems to report to the healthcare provider.
- Follow-up examinations. The importance of follow-up examinations and treatment is stressed to the patient and family because of changing physical status and renal functions.
The focus of documentation in a patient with ARF include:
- Vital signs.
- Muscle strength and reflexes.
- Results of laboratory tests and diagnostic studies.
- Degree of deficit and current sources of fluid intake.
- I&O and fluid balance.
- Plan of care.
- Teaching plan.
- Client’s responses to treatment, teaching, and actions performed.
- Attainment or progress towards the desired outcomes.
- Modifications to plan of care.
- Long term needs.
Posts related to this care plan:
- 6 Acute Renal Failure Nursing Care Plans
- Chronic Renal Failure
- 11 Chronic Renal Failure Nursing Care Plans
- Renal Disorders Nursing Management NCLEX Practice Quiz 1 (50 Items)
11 thoughts on “Acute Renal Failure”
Good information and content
Please provide examples of such patient cases with a proper care plan. THANK YOU
Check out our nursing care plan section.
Great information. Thank you!
Very informative and easy to understand
How can I get more AKI practice questions please?
Hi SF, Great to see your enthusiasm for AKI practice questions! Currently, we have a set of questions available on our website available here: Urinary Disorders NCLEX Practice Quiz (150 Questions)
Great topic. Thank you for the information above.
Hi Graciela, Glad you liked the piece on acute renal failure! 🌟 Any specific parts you found super useful or anything else you’re curious about in this area?
Thank you so much for all the great info. I truly appreciate it.
Leave a Comment Cancel reply
Case Study: An Elderly Patient With Impaired Renal Function
—this case shows the importance of re-evaluating treatment regimens to address adverse effects without exacerbating other comorbidities..
By Emily Donovan Reviewed by Janet B. McGill, MD
This case presents an elderly man with type 2 diabetes and impaired renal function. He has recently been experiencing hypoglycemia with his current treatment regimen. The importance of re-evaluating treatment regimens to address adverse effects without exacerbating other comorbidities, such as renal dysfunction, is discussed.
Case presentation and patient assessment

An 84-year-old man with type 2 diabetes is admitted to the hospital with altered mental status and hypoglycemia. He had experienced recurring episodes of mild hypoglycemia over the previous week, which were managed with food. With this episode, however, he could not be aroused, so the family called EMS. His past medical history includes chronic lymphocytic leukemia, gout, hypertension, and atrial fibrillation. Approximately 6 weeks ago, his serum creatinine increased from 1.6 mg/dL to 2.4 mg/dL following a bout of pneumonia. He had been taking glimepiride 4 mg twice daily. He admitted that he sometimes skipped the dose entirely because of hypoglycemia, and when he did so, his glucose was >200 mg/dL. His glycosylated hemoglobin (HbA1c) is 6.9%. Information and laboratory values collected at the hospital are as follows:
- Height, 5’9”
- Weight, 198 lb
- BP, 114/73 mm Hg
- Current medications, glimepiride 4 mg BID and atorvastatin 10 mg QD
- HbA1c, 6.9%
- Lipids, LDL-C 45 mg/dL; HDL-C 55 mg/dL; Total-C 109 mg/dL; triglycerides 47 mg/dL
- Serum creatinine 1.94 mg/dL
The patient is found to have acceptable glycemic control, but unacceptable episodes of severe hypoglycemia. His worsening renal dysfunction and poor overall health status were likely responsible for the hypoglycemia, since he had tolerated glimepiride in the past. Hypoglycemia with his current treatment regimen was a particular concern given his age, concomitant renal disease, and other comorbidities.
While in the hospital, the patient required a small dose of insulin glargine to control his blood glucose, 16 units.
This patient is elderly and has other comorbidities, including moderate renal impairment. Developing a treatment plan that helps maintain normal glucose levels with little or no associated hypoglycemia as well as limited complexity is important for this elderly patient. 1 Since this patient has moderate renal impairment, it is important to consider medications that are not cleared renally and do not require dose adjustment based on serum creatinine or kidney function. 1 Lastly, renal dysfunction and advanced age are two key risk factors for hypoglycemia. 1-3
At discharge, the decision was made to discontinue insulin glargine and initiate oral therapy with a dipeptidyl peptidase-4 inhibitor, given the low rates of hypoglycemia associated with this class and ease of use (ie, oral administration). 1
Linagliptin 5 mg/day was prescribed at hospital discharge, and a follow-up visit was scheduled in 2 weeks. At the follow-up appointment, the patient reported that he was feeling well and eating better. He stated that his glucose ranged from 140 mg/dL to 210 mg/dL. His serum creatinine had increased to 2.4 mg/dL. He has had no hypoglycemic episodes since leaving the hospital. At this time, a small dose of insulin glargine was added to his glucose-lowering regiment (8 units), with adjustments made on an outpatient basis.
At a 6-month follow-up, the patient’s HbA1c was 6.6%. He reported that his glucose ranges from 110 mg/dL to 155 mg/dL, and he had not experienced any hypoglycemia. His serum creatinine was 1.99 mg/dL. Information and laboratory values collected during the 6-month follow-up visit are summarized here:
- Weight, 186 lb
- BP, 112/70 mm Hg
- Current medications, linagliptin 5 mg/d; insulin glargine 10-14 units/day; atorvastatin 10 mg QD
- HbA1c, 6.6%
- Lipids, LDL-C 28 mg/dL; HDL-C 38 mg/dL; Total-C 103 mg/dL; triglycerides 73 mg/dL
- Serum creatinine 1.99 mg/dL
Over the next 2 years, the patient’s serum creatinine fluctuated from 1.7 mg/dL to 2.5 mg/dL. He required additional chemotherapy for chronic lymphocytic leukemia with small cell lymphoma. His diabetes remained stable on the same regimen of linagliptin 5 mg/day and insulin glargine 10 to 14 units/day.
This case highlights the importance of re-evaluating treatment regimens in elderly patients with renal impairment and hypoglycemia. The 2012 position statement of the American Diabetes Association and the European Association for the Study of Diabetes emphasizes the importance of a patient-centered approach to the treatment of diabetes and, in particular, the need to individualize treatment regimens based on a particular patient’s comorbidities. 1 The American Diabetes Association and the European Association for the Study of Diabetes recommendations state that glycemic targets in elderly patients may need to be less ambitious than those for younger patients with fewer comorbidities and less complicated disease. Furthermore, glucose-lowering regimens for elderly patients should focus on agents that protect against hypoglycemia, renal dysfunction, and drug interactions since the risk of all of these complications is increased in elderly patients. 1 This case illustrates the importance of adjusting a treatment regimen to address a patient’s age, kidney function, and risk of hypoglycemia.
The main goal of therapy for this patient is to achieve glycemic control and minimize the risk of hypoglycemia. In addition, it was important to develop a regimen that did not require dose adjustments for fluctuating kidney function and had a low likelihood of drug interactions with therapies given for other disorders. This patient had numerous comorbidities, and had several additional medications added over the following year to treat his gout, chronic lymphocytic leukemia, and various infections. He remained on the regimen of linagliptin 5 mg/day and insulin glargine, which provided excellent and durable control of his glucose levels with rare episodes of mild hypoglycemia. Providing glucose-lowering therapy with a low propensity for drug interactions in this patient who had multiple comorbidities and was receiving numerous medications that changed over time was also an important aspect of his treatment regimen.
Linagliptin is a rational therapeutic choice for this patient given its primarily nonrenal route of elimination, limited drug interactions, and low risk of hypoglycemia. 4,5 Linagliptin does not require dose adjustment in patients with renal impairment, and has a low likelihood of hypoglycemia in all patients, which is of particular importance in the elderly. 4 The fact that linagliptin is not cleared renally is especially important since impaired renal function can affect drug metabolism (eg, increase concentrations) and predispose patients with renal dysfunction to hypoglycemia. 2 The other DPP-4 inhibitors (alogliptin, saxagliptin, sitagliptin, and vildagliptin) are all predominantly eliminated via renal excretion, and each requires dose adjustment in patients with renal impairment. 1,6
It is also important to note that more severe renal impairment can result in slower elimination of insulin; therefore, dose titration of insulin in patients with severe renal impairment should be done cautiously with recognition for the potential of a more prolonged activity profile in these patients. 1
Post-hoc analyses from the phase III clinical program of linagliptin in patients with type 2 diabetes have shown that it is associated with neutral or favorable effects (on top of standard of care) on renal outcomes as well as consistent glucose-lowering efficacy and tolerability in patients with a range of kidney function. 7-9 Two recent, prospective studies have shown that linagliptin was associated with clinically meaningful improvements in glycemic control and neutral effects on renal parameters in patients with type 2 diabetes and with moderate-to severe renal impairment as well as those with type 2 diabetes and severe renal impairment. 10,11 Overall, these analyses indicate that linagliptin is a safe and effective treatment option in patients with renal impairment.
Elderly patients with type 2 diabetes are at increased risk of hypoglycemia and its consequences. Renal impairment, often present in elderly patients with type 2 diabetes, further increases the risk of hypoglycemia, because it can affect the metabolism of glucose-lowering medications. Therefore, it is important to re-evaluate and adjust treatment regimens for type 2 diabetes in elderly patients with renal impairment to ensure risk of hypoglycemia is minimized and the glucose-lowering regimen does not require dose adjustment for renal impairment. Treatment with linagliptin is a logical choice for elderly patients with renal impairment since it has a predominantly nonrenal route of clearance and low risk of hypoglycemia. In addition, linagliptin does not require dose adjustment for elderly patients or for those with renal impairment, thereby minimizing the complexity of the glucose-lowering regimen in a patient with multiple comorbidities.
Published: November 16, 2016
- 1. Inzucchi SE, Bergenstal RM, Buse JB, et al; American Diabetes Association (ADA); European Association for the Study of Diabetes (EASD). Management of hyperglycemia in type 2 diabetes: a patient-centered approach: position statement of the American Diabetes Association (ADA) and the European Association for the Study of Diabetes (EASD). Diabetes Care . 2012:35:1364-1379
- 2. Moghissi E. Management of type 2 diabetes mellitus in older patients: current and emerging treatment options. Diabetes Ther . 2013;4:239-256.DS) Group. JAMA . 1999;281:2005-2012.
- 3. Ahrén B. Avoiding hypoglycemia: a key to success for glucose-lowering therapy in type 2 diabetes. Vasc Health Risk Manag . 2013;9:155-163.
- 4. McKeage K. Linagliptin: an update of its use in patients with type 2 diabetes mellitus. Drugs . 2014;74:1927-1946.
- 5. Doupis J. Linagliptin: from bench to bedside. Drug Des Devel Ther . 2014;8:431-443.
- 6. Giorda C, Nada E, Tartaglino B. Pharmacokinetics, safety, and efficacy of DPP-4 inhibitors and GLP-1 receptor agonists in patients with type 2 diabetes mellitus and renal or hepatic impairment. A systematic review of the literature. Endocrine . 2014;46:406-419.
- 7. Groop P-H, Cooper ME, Perkovic V, Emser A, Woerle H-J, von Eynatten M. Linagliptin lowers albuminuria on top of recommended standard treatment in patients with type 2 diabetes and renal dysfunction. Diabetes Care . 2013;36:3460-3468.
- 8. Groop PH, Del Prato S, Taskinen MR, et al. Linagliptin treatment in subjects with type 2 diabetes with and without mild-to-moderate renal impairment. Diabetes Obes Metab . 2014;16:560-568
- 9. McGill JB, Barnett AH, Lewin AJ, et al. Linagliptin added to sulphonylurea in uncontrolled type 2 diabetes patients with moderate-to-severe renal impairment. Diab Vasc Dis Res . 2014;11:34-40.
- 10. Laakso M, Rosenstock J, Groop PH, et al. Treatment with the dipeptidyl peptidase-4 inhibitor linagliptin or placebo followed by glimepiride in patients with type 2 diabetes with moderate to severe renal impairment: a 52-week, randomized, double-blind clinical trial. Diabetes Care . 2015;38:e15-e17.
- 11. McGill JB, Sloan L, Newman J, et al. Long-term efficacy and safety of linagliptin in patients with type 2 diabetes and severe renal impairment: a 1-year, randomized, double-blind, placebo-controlled study. Diabetes Care . 2013;36:237-244.
Dpp-4: the physiology of incretin degradation.

Incretins and More: The Physiology of DPP-4
New approaches for dkd in adolescents with t2d.

How Effective is Weight Loss as Therapy for Type 2 Diabetes?

T2D In Youth

Diet in Diabetes: One Size Doesn’t Fit All

Potential Risks of Anti-obesity Medications in Patients with T2D

Treating the Root Cause: Diabetes Medicine


Normal renal MAG3 nuclear study
Citation, doi, disclosures and case data.
At the time the case was submitted for publication Craig Hacking had no recorded disclosures.
Presentation
Recurrent flank pain. Recurrent left PUJ obstruction. Pyeloplasty 7 years earlier, known capacious left renal pelvis.
Patient Data
Tc99m-MAG3 was administered via IV cannula.IV frusemide was administered immediately prior to the imaging protocol start. Dynamic and planar imaging was performed.
Kidneys are in normal position. Normal symmetric renal perfusion bilaterally. Normal bilateral cortical radiotracer uptake. Bilateral central photopenia secondary to prominent renal pelvis, more on the left.
Radiotracer demonstrated within the renal pelvis by 60 seconds. The left renal pelvis is slightly prominent compared to the right.
Normal emptying of radiotracer from the upper urinary tract and ureters.
Normal frusemide clearance half times of 7 minutes on the left, and 4 minutes on the right (normal < 10 minutes).
The differential renal function at 2-3 minutes is symmetrical at 50% on the left and 50% on the right.
- No functionally significant renal outflow tract obstruction.
- Normal split renal function of 50% on each side.
Case Discussion
MAG3 radiotracer has tubular excretion in the kidney and is used as a test for renal tract obstruction. This is a case of a normal MAG3 study.
1 article features images from this case
- Tc-99m MAG3
9 public playlists include this case
- Uro teaching 7-4-2022 by Salman Atiq Siddiqui
- Nuclear Medicine by Lim Yi Shan May
- Nuclear Medicine Tutorial 2 by Amit Parekh
- Viva by HYDER ALI
- 2B: GU by Daoud akhtar
- 2B: Nuc Med by Daoud akhtar
- Urology CIM exam by Sachinka Ranasinghe
- مهم by majid alipoor
- nuc med mar 2024 by Soumya Cicilet
Related Radiopaedia articles
- Normal genitourinary tract imaging examples
Promoted articles (advertising)
How to use cases.
You can use Radiopaedia cases in a variety of ways to help you learn and teach.
- Add cases to playlists
- Share cases with the diagnosis hidden
- Use images in presentations
- Use them in multiple choice question
Creating your own cases is easy.
- Case creation learning pathway
ADVERTISEMENT: Supporters see fewer/no ads
By Section:
- Artificial Intelligence
- Classifications
- Imaging Technology
- Interventional Radiology
- Radiography
- Central Nervous System
- Gastrointestinal
- Gynaecology
- Haematology
- Head & Neck
- Hepatobiliary
- Interventional
- Musculoskeletal
- Paediatrics
- Not Applicable
Radiopaedia.org
- Feature Sponsor
- Expert advisers

- Open access
- Published: 10 January 2022
Chronic kidney disease and its health-related factors: a case-control study
- Mousa Ghelichi-Ghojogh 1 ,
- Mohammad Fararouei 2 ,
- Mozhgan Seif 3 &
- Maryam Pakfetrat 4
BMC Nephrology volume 23 , Article number: 24 ( 2022 ) Cite this article
19k Accesses
10 Citations
8 Altmetric
Metrics details
Chronic kidney disease (CKD) is a non-communicable disease that includes a range of different physiological disorders that are associated with abnormal renal function and progressive decline in glomerular filtration rate (GFR). This study aimed to investigate the associations of several behavioral and health-related factors with CKD in Iranian patients.
A hospital-based case-control study was conducted on 700 participants (350 cases and 350 controls). Logistic regression was applied to measure the association between the selected factors and CKD.
The mean age of cases and controls were 59.6 ± 12.4 and 58.9 ± 12.2 respectively ( p = 0.827). The results of multiple logistic regression suggested that many factors including low birth weight (OR yes/no = 4.07, 95%CI: 1.76–9.37, P = 0.001), history of diabetes (OR yes/no = 3.57, 95%CI: 2.36–5.40, P = 0.001), history of kidney diseases (OR yes/no = 3.35, 95%CI: 2.21–5.00, P = 0.001) and history of chemotherapy (OR yes/no = 2.18, 95%CI: 1.12–4.23, P = 0.02) are associated with the risk of CKD.
Conclusions
The present study covered a large number of potential risk/ preventive factors altogether. The results highlighted the importance of collaborative monitoring of kidney function among patients with the above conditions.
Peer Review reports
Chronic kidney disease (CKD) is a non-communicable disease that includes a range of different physiological disorders that are associated with an abnormal renal function and progressive decline in glomerular filtration rate (GFR) [ 1 , 2 , 3 ]. Chronic kidney disease includes five stages of kidney damage, from mild kidney dysfunction to complete failure [ 4 ]. Generally, a person with stage 3 or 4 of CKD is considered as having moderate to severe kidney damage. Stage 3 is broken up into two levels of kidney damage: 3A) a level of GFR between 45 to 59 ml/min/1.73 m 2 , and 3B) a level of GFR between 30 and 44 ml/min/1.73 m 2 . In addition, GFR for stage 4 is 15–29 ml/min/1.73 m 2 [ 4 , 5 ]. It is reported that both the prevalence and burden of CKD are increasing worldwide, especially in developing countries [ 6 ]. The worldwide prevalence of CKD (all stages) is estimated to be between 8 to 16%, a figure that may indicate millions of deaths annually [ 7 ]. According to a meta-analysis, the prevalence of stage 3 to 5 CKD in South Africa, Senegal, and Congo is about 7.6%. In China, Taiwan, and Mongolia the rate of CKD is about 10.06% and in Japan, South Korea, and Oceania the rate is about 11.73%. In Europe the prevalence of CKD is about 11.86% [ 8 ], and finally, about 14.44% in the United States and Canada. The prevalence of CKD is estimated to be about 11.68% among the Iranian adult population and about 2.9% of Iranian women and 1.3% of Iranian men are expected to develop CKD annually [ 9 ]. Patients with stages 3 or 4 CKD are at much higher risk of progressing to either end-stage renal disease (ESRD) or death even prior to the development of ESRD [ 10 , 11 ].
In general, a large number of risk factors including age, sex, family history of kidney disease, primary kidney disease, urinary tract infections, cardiovascular disease, diabetes mellitus, and nephrotoxins (non-steroidal anti-inflammatory drugs, antibiotics) are known as predisposing and initiating factors of CKD [ 12 , 13 , 14 ]. However, the existing studies are suffering from a small sample size of individuals with kidney disease, particularly those with ESRD [ 15 ].
Despite the fact that the prevalence of CKD in the world, including Iran, is increasing, the factors associated with CKD are explored very little. The present case-control study aimed to investigate the association of several behavioral and health-related factors with CKD in the Iranian population.
Materials and methods
In this study, participants were selected among individuals who were registered or were visiting Faghihi and Motahari hospitals (two largest referral centers in the South of Iran located in Shiraz (the capital of Fars province). Cases and controls were frequency-matched by sex and age. The GFR values were calculated using the CKD-EPI formula [ 16 , 17 ].
Data collection
An interview-administered questionnaire and the participant’s medical records were used to obtain the required data. The questionnaire and interview procedure were designed, evaluated, and revised by three experts via conducting a pilot study including 50 cases and 50 controls. The reliability of the questionnaire was measured using the test-retest method (Cronbach’s alpha was 0.75). The interview was conducted by a trained public health nurse at the time of visiting the clinics.
Avoiding concurrent conditions that their association may interpreted as reverse causation; the questionnaire was designed to define factors preceding at least a year before experiencing CKD first symptoms. Accordingly participants reported their social and demographic characteristics (age, sex, marital status, educational level, place of residency), history of chronic diseases (diabetes, cardiovascular diseases, hypertension, kidney diseases, family history of kidney diseases, autoimmune diseases and thyroid diseases [ 18 ]). Also history of other conditions namely (smoking, urinary tract infection (UTI), surgery due to illness or accident, low birth weight, burns, kidney pain (flank pain), chemotherapy, taking drugs for weight loss or obesity, taking non-steroidal anti-inflammatory drugs, and taking antibiotic) before their current condition was started. Many researchers reported recalling birth weight to be reliable for research purposes [ 19 ]. Moreover, we asked the participants to report their birth weight as a categorical variable (< 2500 g or low, 2500- < 3500 g or normal, and > 3500 g or overweight). Medical records of the participants were used to confirm/complete the reported data. In the case of contradiction between the self-reported and recorded data, we used the recorded information for our study.
Verbal informed consent was obtained from patients because the majority of the participants were illiterate. The study protocol was reviewed and approved by the ethical committee of Shiraz University of Medical Sciences (approval number: 1399.865).
Sample size
The sample size was calculated to detect an association between the history of using antibiotics (one of our main study variables) and CKD as small as OR = 1.5 [ 20 ]. With an alpha value of 0.05 (2-sided) and a power of 80%, the required sample size was estimated as large as n = 312 participants for each group.
Selection of cases
The selected clinics deliver medical care to patients from the southern part of the country. In this study, patients with CKD who were registered with the above centers from June to December 2020 were studied. A case was a patient with a GFR < 60 (ml/min/1.73 m 2 ) at least twice in 3 months. According to the latest version of the International Classification of Diseases (2010), Codes N18.3 and N18.4 are assigned to patients who have (GFR = 30–59 (ml/min/1.73 m 2 ) and GFR = 15–29 (ml/min/1.73 m 2 ) respectively [ 21 ]. In total, 350 patients who were diagnosed with CKD by a nephrologist during the study period.
Selection of the controls
We used hospital controls to avoid recall-bias. The control participants were selected from patients who were admitted to the general surgery (due to hernia, appendicitis, intestinal obstruction, hemorrhoids, and varicose veins), and orthopedic wards from June to December 2020. Using the level of creatinine in the participants’ serum samples, GFR was calculated and the individuals with normal GFR (ml/min/1.73 m 2 ) GFR > 60) and those who reported no history of CKD were included ( n = 350).
Inclusion criteria
Patients were included if they were ≥ 20 years old and had a definitive diagnosis of CKD by a nephrologist.
Exclusion criteria
Participants were excluded if they were critically ill, had acute kidney injury, those undergone renal transplantation, and those with cognitive impairment.
Statistical analysis
The Chi-square test was used to measure the unadjusted associations between categorical variables and CKD. Multiple logistic regression was applied to measure the adjusted associations for the study variables and CKD. The backward variable selection strategy was used to include variables in the regression model. Odds ratios (ORs) and 95% confidence intervals (CIs) were calculated. All p -values were two-sided and the results were considered statistically significant at p < 0.05. All analyses were conducted using Stata version 14.0 (Stata Corporation, College Station, TX, USA).
In total, 350 cases and 350 age and sex-matched controls were included in the analysis. The mean age of cases and controls were 59.6 ± 12.4 and 58.9 ± 12.2 respectively ( p = 0.83). Overall, 208 patients (59.4%) and 200 controls (57.1%) were male ( p = 0.54). Also, 149 patients (42.6%) and 133 controls (38.0%) were illiterate or had elementary education ( p = 0.001). Most cases (96.9%) and controls (95.7%) were married ( p = 0.42). The mean GFR for CKD and control groups were 38.6 ± 11.4 and 78.3 ± 10.2 (ml/min/1.73 m2) respectively.
Result of univariate analysis
Table 1 illustrates the unadjusted associations of demographic and health-related variables with CKD. Accordingly, significant (unadjusted) associations were found between the risk of CKD and several study variables including education, history of chronic diseases (diabetes, cardiovascular, hypertension, kidney diseases, autoimmune diseases, and hypothyroidism), family history of kidney diseases, smoking, UTI, surgery due to illness or accident, low birth weight, burns, kidney pain, chemotherapy, taking non-steroidal anti-inflammatory drugs, and taking antibiotics) ( P < 0.05 for all).
Results of multivariable analysis
Table 2 illustrates the adjusted associations between the study variables and the risk of CKD. Most noticeably, low birth weight (OR yes/no = 4.07, 95%CI: 1.76–9.37, P = 0.001), history of surgery (OR yes/no = 1.74, 95%CI: 1.18–2.54, P = 0.004), family history of kidney diseases (OR yes/no = 1.97, 95%CI: 1.20–3.23, P = 0.007), and history of chemotherapy (OR yes/no = 2.18, 95%CI: 1.12–4.23, P = 0.02) were significantly associated with a higher risk of CKD. On the other hand, education (OR college/illiterate or primary = 0.54, 95%CI: 0.31–0.92, P = 0.025) was found to be inversely associated with CKD.
The results of the present study suggested that several variables including, education, history of diabetes, history of hypertension, history of kidney diseases or a family history of kidney diseases, history of surgery due to illness or accident, low birth weight, history of chemotherapy, history of taking non-steroidal anti-inflammatory drugs, and history of taking antibiotics may affect the risk of CKD.
In our study, the level of education was inversely associated with the risk of CKD. This finding is in accordance with the results of a study conducted by K Lambert et.al, who suggested that illiteracy or elementary education may raise the risk of CKD [ 22 ]. The fact that education level is associated with health literacy, may partly explain our results that lower education and inadequate health literacy in individuals with CKD is associated with worse health outcomes including poorer control of biochemical parameters, higher risk of cardiovascular diseases (CVDs); a higher rate of hospitalization, and a higher rate of infections [ 23 ].
In the current study, the history of diabetes was associated with a higher risk of CKD. This finding is consistent with the results of other studies on the same subject [ 20 , 21 , 24 , 25 , 26 , 27 ]. It is not surprising that people with diabetes have an increased risk of CKD as diabetes is an important detrimental factor for kidney functioning as approximately, 40% of patients with diabetes develop CKD [ 27 ].
The other variable that was associated with an increased risk of CKD was a history of hypertension. Our result is consistent with the results of several other studies [ 20 , 24 , 25 , 28 ]. It is reported that hypertension is both a cause and effect of CKD and accelerates the progression of the CKD to ESRD [ 29 ].
After controlling for other variables, a significant association was observed between family history of kidney diseases and risk of CKD. Published studies suggested the same pattern [ 24 ]. Inherited kidney diseases (IKDs) are considered as the foremost reasons for the initiation of CKD and are accounted for about 10–15% of kidney replacement therapies (KRT) in adults [ 30 ].
The importance of the history of surgery due to illness or accident in this study is rarely investigated by other researchers who reported the effect of surgery in patients with acute kidney injury (AKI), and major abdominal and cardiac surgeries [ 31 , 32 ] on the risk of CKD. Also, AKI is associated with an increased risk of CKD with progression in various clinical settings [ 33 , 34 , 35 ]. In a study by Mizota et.al, although most AKI cases recovered completely within 7 days after major abdominal surgery, they were at higher risk of 1-year mortality and chronic kidney disease compared to those without AKI [ 31 ].
The present study also showed that low birth weight is a significant risk factor for CKD. This finding is consistent with the results of some other studies. However, the results of very few studies on the association between birth weight and risk of CKD are controversial as some suggested a significant association [ 19 , 36 , 37 ] whereas others suggested otherwise [ 36 ]. This may be explained by the relatively smaller size and volume of kidneys in LBW infants compared to infants that are normally grown [ 38 ]. This can lead to long-term complications in adolescence and adulthood including hypertension, decreased glomerular filtration, albuminuria, and cardiovascular diseases. Eventually, these long-term complications can also cause CKD [ 39 ].
Another important result of the current study is the association between chemotherapy for treating cancers and the risk of CKD. According to a study on chemotherapy for testicular cancer by Inai et al., 1 year after chemotherapy 23% of the patients showed CKD [ 40 ]. Another study suggested that the prevalence of stage 3 CKD among patients with cancer was 12, and < 1% of patients had stage 4 CKD [ 41 , 42 ]. Other studies have shown an even higher prevalence of CKD among cancer patients. For instance, only 38.6% of patients with breast cancer, 38.9% of patients with lung cancer, 38.3% of patients with prostate cancer, 27.5% of patients with gynecologic cancer, and 27.2% of patients with colorectal cancer had a GFR ≥90 (ml/min/1.73 m 2 ) at the time of therapy initiation [ 43 , 44 ]. The overall prevalence of CKD ranges from 12 to 25% across many cancer patients [ 45 , 46 , 47 ]. These results clearly demonstrate that, when patients with cancer develop acute or chronic kidney disease, outcomes are inferior, and the promise of curative therapeutic regimens is lessened.
In our study, the history of taking nephrotoxic agents (antibiotics or NSAIDs drugs) was associated with a higher risk of CKD. Our result is following the results reported by other studies [ 48 , 49 ]. Common agents that are associated with AKI include NSAIDs are different drugs including antibiotics, iodinated contrast media, and chemotherapeutic drugs [ 50 ].
Strengths and limitations of our study
Our study used a reasonably large sample size. In addition, a considerably large number of study variables was included in the study. With a very high participation rate, trained nurses conducted the interviews with the case and control participants in the same setting. However, histories of exposures are prone to recall error (bias), a common issue in the case-control studies. It is to be mentioned that the method of selecting controls (hospital controls) should have reduced the risk of recall bias when reporting the required information. In addition, we used the participants’ medical records to complete/ confirm the reported data. Although the design of the present study was not able to confirm a causal association between the associated variables and CKD, the potential importance and modifiable nature of the associated factors makes the results potentially valuable and easily applicable in the prevention of CKD.
Given that, chemotherapy is an important risk factor for CKD, we suggest the imperative for collaborative care between oncologists and nephrologists in the early diagnosis and treatment of kidney diseases in patients with cancer. Training clinicians and patients are important to reduce the risk of nephrotoxicity. Electronic medical records can simultaneously be used to monitor prescription practices, responsiveness to alerts and prompts, the incidence of CKD, and detecting barriers to the effective implementation of preventive measures [ 51 ]. Routine follow-up and management of diabetic patients is also important for the prevention of CKD. We suggest a tight collaboration between endocrinologists and nephrologists to take care of diabetic patients with kidney problems. In addition, surgeons in major operations should refer patients, especially patients with AKI, to a nephrologist for proper care related to their kidney function. Treatment of hypertension is among the most important interventions to slow down the progression of CKD [ 12 ]. Moreover, all patients with newly diagnosed hypertension should be screened for CKD. We suggest all patients with diabetes have their GFR and urine albumin-to-creatinine ratio (UACR) checked annually. Finally, the aging population and obesity cause the absolute numbers of people with diabetes and kidney diseases to raise significantly. This will require a more integrated approach between dialectologists/nephrologists and the primary care teams (55).
Availability of data and materials
The datasets generated and/or analyzed during the current study are not publicly available due to their being the intellectual property of Shiraz University of Medical Sciences but are available from the corresponding author on reasonable request.
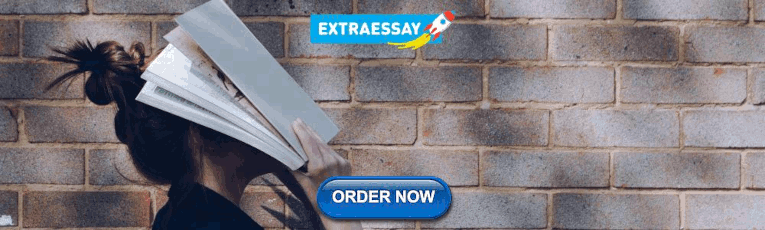
Abbreviations
- Chronic kidney disease
End-stage renal disease
Glomerular filtration rate
Renal replacement treatment
Urinary tract infection
Odds ratios
Confidence intervals
Hypertension
Acute kidney injury
Ghelichi Ghojogh M, Salarilak S, Taghezadeh Afshari A, Khalkhali HR, Mohammadi-Fallah MR, Mkhdoomi K. The effect of body mass index on patient and graft survival rate in kidney transplanted patients in Iran. Nephrourol Monthly. 2017;9(4):e14386.
Zeba Z, Fatema K, Sumit AF, Zinnat R, Ali L. Early screening of chronic kidney disease patients among the asymptomatic adult population in Bangladesh. J Prev Epidemiol. 2020;5(1):e10–e.
Article Google Scholar
Mahajan C, Tiwari V, Divyaveer SS, Patil MR, Banerjee A, Bagur V, et al. Spectrum of renal biopsies; a three-year data from a tertiary care Centre of eastern India. J Nephropharmacol. 2020;9(2):e20–e.
Article CAS Google Scholar
Kimura K, Hosoya T, Uchida S, Inaba M, Makino H, Maruyama S, et al. Febuxostat therapy for patients with stage 3 CKD and asymptomatic Hyperuricemia: a randomized trial. Am J Kidney Dis. 2018;72(6):798–810.
Article CAS PubMed Google Scholar
Foster MC, Hwang S-J, Larson MG, Lichtman JH, Parikh NI, Vasan RS, et al. Overweight, obesity, and the development of stage 3 CKD: the Framingham heart study. Am J Kidney Dis. 2008;52(1):39–48.
Article PubMed PubMed Central Google Scholar
Rachmi CN, Agho KE, Li M, Baur LA. Stunting, underweight and overweight in children aged 2.0–4.9 years in Indonesia: prevalence trends and associated risk factors. PLoS One. 2016;11(5):e0154756.
Asghari G, Momenan M, Yuzbashian E, Mirmiran P, Azizi F. Dietary pattern and incidence of chronic kidney disease among adults: a population-based study. Nutr Metab. 2018;15(1):1–11.
Ruggles DR, Freyman RL, Oxenham AJ. Influence of musical training on understanding voiced and whispered speech in noise. PLoS One. 2014;9(1):e86980.
Moazzeni SS, Arani RH, Hasheminia M, Tohidi M, Azizi F, Hadaegh F. High incidence of chronic kidney disease among Iranian diabetic adults: using CKD-EPI and MDRD equations for estimated glomerular filtration rate. Korean Diabetes J. 2021;45(5):684-97.
Salam SN, Eastell R, Khwaja A. Fragility fractures and osteoporosis in CKD: pathophysiology and diagnostic methods. Am J Kidney Dis. 2014;63(6):1049–59.
Zahmatkesh M, Tamadon MR. World kidney day 2018; chronic kidney disease in women. J Nephropathol. 2017;7(1):4–6.
Noble R, Taal MW. Epidemiology and causes of chronic kidney disease. Medicine. 2019;47(9):562–6.
Lopez-Garcia E, Schulze MB, Fung TT, Meigs JB, Rifai N, Manson JE, et al. Major dietary patterns are related to plasma concentrations of markers of inflammation and endothelial dysfunction. Am J Clin Nutr. 2004;80(4):1029–35.
Sepahi MA, Niknafs B. Multifaceted role of apolipoprotein L1 risk variants and nephropathy. J Nephropathol. 2020;9(1):1-3.
Cohen JB, Tewksbury CM, Landa ST, Williams NN, Dumon KR. National postoperative bariatric surgery outcomes in patients with chronic kidney disease and end-stage kidney disease. Obes Surg. 2019;29(3):975–82.
Levey AS, Andreoli SP, DuBose T, Provenzano R, Collins AJ. Chronic kidney disease: common, harmful and treatable–world kidney day 2007. Am J Nephrol. 2007;27(1):108–12.
Article PubMed Google Scholar
Argulian E, Sherrid MV, Messerli FH. Misconceptions and facts about hypertrophic cardiomyopathy. Am J Med. 2016;129(2):148–52.
Rhee CM. The interaction between thyroid and kidney disease: an overview of the evidence. Curr Opin Endocrinol Diabetes Obes. 2016;23(5):407.
Article CAS PubMed PubMed Central Google Scholar
Al Salmi I, Hoy WE, Kondalsamy-Chennakes S, Wang Z, Healy H, Shaw JE. Birth weight and stages of CKD: a case-control study in an Australian population. Am J Kidney Dis. 2008;52(6):1070–8.
Yacoub R, Habib H, Lahdo A, Al Ali R, Varjabedian L, Atalla G, et al. Association between smoking and chronic kidney disease: a case control study. BMC Public Health. 2010;10(1):1–6.
Saucier NA, Sinha MK, Liang KV, Krambeck AE, Weaver AL, Bergstralh EJ, et al. Risk factors for CKD in persons with kidney stones: a case-control study in Olmsted County, Minnesota. Am J Kidney Dis. 2010;55(1):61–8.
Lambert K, Mullan J, Mansfield K, Lonergan M. A cross-sectional comparison of health literacy deficits among patients with chronic kidney disease. J Health Commun. 2015;20(sup2):16–23.
Fraser SD, Roderick PJ, Casey M, Taal MW, Yuen HM, Nutbeam D. Prevalence and associations of limited health literacy in chronic kidney disease: a systematic review. Nephrol Dial Transplant. 2013;28(1):129–37.
Ji MY, Park YS, Yi SE. A case-control study to identify the risk factors of school accidents. Korean J Epidemiol. 2005;27(2):80–94.
Google Scholar
Khajehdehi P, Malekmakan L, Pakfetrat M, Roozbeh J, Sayadi M. Prevalence of chronic kidney disease and its contributing risk factors in southern Iran a cross-sectional adult population-based study; 2014.
Li H, Lu W, Wang A, Jiang H, Lyu J. Changing epidemiology of chronic kidney disease as a result of type 2 diabetes mellitus from 1990 to 2017: estimates from global burden of disease 2017. J Diabetes Investig. 2021;12(3):346.
Xu Y, Surapaneni A, Alkas J, Evans M, Shin J-I, Selvin E, et al. Glycemic control and the risk of acute kidney injury in patients with type 2 diabetes and chronic kidney disease: parallel population-based cohort studies in US and Swedish routine care. Diabetes Care. 2020;43(12):2975–82.
Sepanlou SG, Barahimi H, Najafi I, Kamangar F, Poustchi H, Shakeri R, et al. Prevalence and determinants of chronic kidney disease in northeast of Iran: results of the Golestan cohort study. PLoS One. 2017;12(5):e0176540.
Pugh D, Gallacher PJ, Dhaun N. Management of hypertension in chronic kidney disease. Drugs. 2019;79(4):365–79.
Torra R, Furlano M, Ortiz A, Ars E. Genetic kidney diseases as an underecognized cause of chronic kidney disease: the key role of international registry reports. Clin Kidney J. 2021;14(8):1879-85.
Mizota T, Dong L, Takeda C, Shiraki A, Matsukawa S, Shimizu S, et al. Transient acute kidney injury after major abdominal surgery increases chronic kidney disease risk and 1-year mortality. J Crit Care. 2019;50:17–22.
Madsen NL, Goldstein SL, Frøslev T, Christiansen CF, Olsen M. Cardiac surgery in patients with congenital heart disease is associated with acute kidney injury and the risk of chronic kidney disease. Kidney Int. 2017;92(3):751–6.
Newsome BB, Warnock DG, McClellan WM, Herzog CA, Kiefe CI, Eggers PW, et al. Long-term risk of mortality and end-stage renal disease among the elderly after small increases in serum creatinine level during hospitalization for acute myocardial infarction. Arch Intern Med. 2008;168(6):609–16.
Ishani A, Nelson D, Clothier B, Schult T, Nugent S, Greer N, et al. The magnitude of acute serum creatinine increase after cardiac surgery and the risk of chronic kidney disease, progression of kidney disease, and death. Arch Intern Med. 2011;171(3):226–33.
James MT, Ghali WA, Knudtson ML, Ravani P, Tonelli M, Faris P, et al. Associations between acute kidney injury and cardiovascular and renal outcomes after coronary angiography. Circulation. 2011;123(4):409–16.
Esmeijer K, de Vries AP, Mook-Kanamori DO, de Fijter JW, Rosendaal FR, Rabelink TJ, et al. Low birth weight and kidney function in middle-aged men and women: the Netherlands epidemiology of obesity study. Am J Kidney Dis. 2019;74(6):751–60.
White SL, Perkovic V, Cass A, Chang CL, Poulter NR, Spector T, et al. Is low birth weight an antecedent of CKD in later life? A systematic review of observational studies. Am J Kidney Dis. 2009;54(2):248–61.
Harer MW, Charlton JR, Tipple TE, Reidy KJ. Preterm birth and neonatal acute kidney injury: implications on adolescent and adult outcomes. J Perinatol. 2020;40(9):1286–95.
Al Salmi I, Hannawi S. Birth weight and susceptibility to chronic kidney disease. Saudi J Kidney Dis Transplant. 2020;31(4):717.
Inai H, Kawai K, Ikeda A, Ando S, Kimura T, Oikawa T, et al. Risk factors for chronic kidney disease after chemotherapy for testicular cancer. Int J Urol. 2013;20(7):716–22.
Launay-Vacher V, Oudard S, Janus N, Gligorov J, Pourrat X, Rixe O, et al. Prevalence of renal insufficiency in cancer patients and implications for anticancer drug management: the renal insufficiency and anticancer medications (IRMA) study. Cancer. 2007;110(6):1376–84.
Launay-Vacher V. Epidemiology of chronic kidney disease in cancer patients: lessons from the IRMA study group. Semin Nephrol. 2010;30(6):548–56.
Launay-Vacher V, Janus N, Deray G. Renal insufficiency and cancer treatments. ESMO Open. 2016;1(4):e000091.
Janus N, Launay-Vacher V, Byloos E, Machiels JP, Duck L, Kerger J, et al. Cancer and renal insufficiency results of the BIRMA study. Br J Cancer. 2010;103(12):1815–21.
Kitchlu A, McArthur E, Amir E, Booth CM, Sutradhar R, Majeed H, et al. Acute kidney injury in patients receiving systemic treatment for Cancer: a population-based cohort study. J Natl Cancer Inst. 2019;111(7):727–36.
Kidney Disease Improving Global Outcomes (KDIGO). KDIGO clinical practice guideline for acute kidney injury. kdigo.org/wpcontent/uploads/2016/10/KDIGO-2012-AKI-Guide line-Engli sh.pdf . Accessed 23 Mar 2020.
Königsbrügge O, Lötsch F, Zielinski C, Pabinger I, Ay C. Chronic kidney disease in patients with cancer and its association with occurrence of venous thromboembolism and mortality. Thromb Res. 2014;134(1):44–9.
Goldstein SL, Mottes T, Simpson K, Barclay C, Muething S, Haslam DB, et al. A sustained quality improvement program reduces nephrotoxic medication-associated acute kidney injury. Kidney Int. 2016;90(1):212–21.
Menon S, Kirkendall ES, Nguyen H, Goldstein SL. Acute kidney injury associated with high nephrotoxic medication exposure leads to chronic kidney disease after 6 months. J Pediatr. 2014;165(3):522–7.e2.
Perazella MA, Izzedine H. New drug toxicities in the onco-nephrology world. Kidney Int. 2015;87(5):909–17.
Luyckx VA, Tuttle KR, Garcia-Garcia G, Gharbi MB, Heerspink HJL, Johnson DW, et al. Reducing major risk factors for chronic kidney disease. Kidney Int Suppl. 2017;7(2):71–87.
Download references
Acknowledgments
This paper is part of a thesis conducted by Mousa Ghelichi-Ghojogh, Ph.D. student of epidemiology, and a research project conducted at the Shiraz University of Medical sciences (99-01-04-22719). We would like to thank Dr. Bahram Shahryari and all nephrologists of Shiraz University of medical sciences, interviewers, and CKD patients in Shiraz for their voluntary participation in the study and for providing data for the study.
Shiraz University of Medical Sciences financially supported this study. (Grant number: 99–01–04-22719).
Author information
Authors and affiliations.
Candidate in Epidemiology, Student Research Committee, Shiraz University of Medical Sciences, Shiraz, Iran
Mousa Ghelichi-Ghojogh
HIV/AIDS research center, School of Health, Shiraz University of Medical Sciences, P.O.Box: 71645-111, Shiraz, Iran
Mohammad Fararouei
Department of Epidemiology, School of Health, Shiraz University of Medical Sciences, Shiraz, Iran
Mozhgan Seif
Nephrologist, Shiraz Nephro-Urology Research Center, Department of Internal Medicine, Emergency Medicine Research Center, Shiraz University of Medical Sciences, Shiraz, Iran
Maryam Pakfetrat
You can also search for this author in PubMed Google Scholar
Contributions
MGG: Conceptualization, Methodology, Statistical analysis, Investigation, and writing the draft of the manuscript. MP: were involved in methodology, writing the draft of the manuscript, and clinical consultation. MS: was involved in the methodology and statistical analysis. MF: was involved in conceptualization, methodology, supervision, writing, and reviewing the manuscript. The authors read and approved the final manuscript.
Corresponding author
Correspondence to Mohammad Fararouei .
Ethics declarations
Ethics approval and consent to participate.
The study protocol was reviewed and approved by the ethical committee of Shiraz University of Medical Sciences (approval number: 1399.865). All methods were performed in accordance with the relevant guidelines and regulations of the Declaration of Helsinki. The participants were assured that their information is used for research purposes only. Because of the illiteracy of a considerable number of the patients, verbal informed consent was obtained from the participants. Using verbal informed consent was also granted by the ethical committee of Shiraz University of Medical Sciences.
Consent for publication
Not applicable.
Competing interests
None of the authors declare disclosures of direct relevance to the submitted work.
Additional information
Publisher’s note.
Springer Nature remains neutral with regard to jurisdictional claims in published maps and institutional affiliations.
Rights and permissions
Open Access This article is licensed under a Creative Commons Attribution 4.0 International License, which permits use, sharing, adaptation, distribution and reproduction in any medium or format, as long as you give appropriate credit to the original author(s) and the source, provide a link to the Creative Commons licence, and indicate if changes were made. The images or other third party material in this article are included in the article's Creative Commons licence, unless indicated otherwise in a credit line to the material. If material is not included in the article's Creative Commons licence and your intended use is not permitted by statutory regulation or exceeds the permitted use, you will need to obtain permission directly from the copyright holder. To view a copy of this licence, visit http://creativecommons.org/licenses/by/4.0/ . The Creative Commons Public Domain Dedication waiver ( http://creativecommons.org/publicdomain/zero/1.0/ ) applies to the data made available in this article, unless otherwise stated in a credit line to the data.
Reprints and permissions
About this article
Cite this article.
Ghelichi-Ghojogh, M., Fararouei, M., Seif, M. et al. Chronic kidney disease and its health-related factors: a case-control study. BMC Nephrol 23 , 24 (2022). https://doi.org/10.1186/s12882-021-02655-w
Download citation
Received : 14 August 2021
Accepted : 24 December 2021
Published : 10 January 2022
DOI : https://doi.org/10.1186/s12882-021-02655-w
Share this article
Anyone you share the following link with will be able to read this content:
Sorry, a shareable link is not currently available for this article.
Provided by the Springer Nature SharedIt content-sharing initiative
- Related factors
- Case-control
BMC Nephrology
ISSN: 1471-2369
- Submission enquiries: [email protected]
- General enquiries: [email protected]

A .gov website belongs to an official government organization in the United States.
A lock ( ) or https:// means you've safely connected to the .gov website. Share sensitive information only on official, secure websites.
- Risk Factors
- Caring for Yourself and Others
- Living with Chronic Kidney Disease
- Chronic Kidney Disease: Common, Serious, and Costly
- About the CKD Initiative
- Chronic Kidney Disease in the United States, 2023
- Chronic Kidney Disease Is Increasing in Mexican American Adults
- Publications
Testing for Chronic Kidney Disease
- If you have diabetes or high blood pressure, you have a higher risk for chronic kidney disease (CKD).
- Having your kidneys checked regularly gives you the best chance for finding and treating CKD early.
- Learn about kidney testing and what your results could mean.
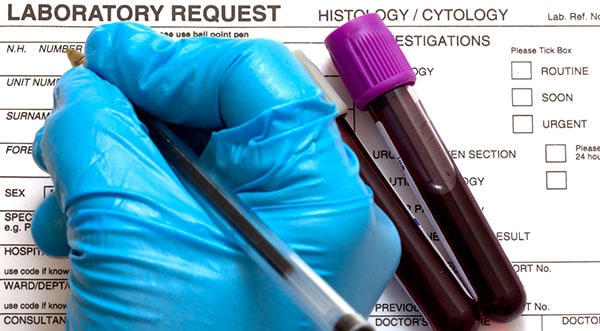
CKD and kidney testing
CKD happens when your kidneys become damaged over time and can't filter your blood as well. Diabetes is a leading cause of CKD, and there are often no symptoms until your kidneys are badly damaged.
If you find and treat kidney disease early, you may be able to manage CKD and prevent other health complications. But the only way to know how well your kidneys are working is to get tested.
Urine tests
One of the earliest signs of kidney disease is when protein leaks into your urine (proteinuria). Urine testing can check for this. There are two types of urine tests that can check your protein levels.
Dipstick urine test. A dipstick is a chemically treated paper placed in your urine sample. It changes color if your levels are above normal. This test is often done as part of overall urine testing. It can also be done as a quick test to look for albumin (a protein produced by your liver) in your urine.
A dipstick urine test doesn't provide an exact measurement of albumin but does let your doctor know if your levels are normal. If you have abnormal albumin levels, your doctor may want to run further tests.
Urine albumin-to-creatinine ratio (UACR). This test measures the amount of albumin and compares it to the amount of creatinine (a normal waste product from your muscles) in your urine. A UACR test lets the doctor know how much albumin passes into your urine over a 24-hour period. A urine albumin test result of 30 or above may mean kidney disease.
It's important to know that:
- The test may be repeated once or twice to confirm the results.
- If you have kidney disease, your albumin level in your urine helps your doctor determine the best treatment option.
- If your urine albumin level stays the same or goes down, it means your treatment is working.
Serum creatinine
Because your kidneys remove waste, toxins, and extra fluid from the blood, blood tests can check your kidney function. They will show how well and how quickly your kidneys are doing their job to remove waste.
A serum creatinine blood test measures the amount of creatinine in your blood. If your kidneys aren't working well, your creatinine level goes up. Normal levels for you will depend on your sex, age, and muscle mass.
Usually a creatinine level more than 1.2 for women and 1.4 for men may mean the kidneys aren't working well.
Glomerular filtration rate (GFR)
The GFR is a blood test that measures how well your kidneys remove waste, toxins, and extra fluid from your blood. Your serum creatinine level, age, and sex are used to calculate your GFR number. Like other kidney tests, a normal GFR number for you will depend on your age and sex.
If your GFR is low, your kidneys are likely not working as they should. As kidney disease progresses, your GFR goes down. The results of your test can mean the following:
If your GFR is 60 or more together with a normal urine albumin test, you are in the normal range. But you'll still want to talk to your doctor about when you should be checked again.
If your GFR is less than 60 , it may mean you have kidney disease. You'll want to talk to your doctor about treatment options that are best for you.
If your GFR is less than 15 , it may mean your kidneys are failing. If your results show kidney failure, you'll likely need dialysis or a kidney transplant. If your GFR level is less than 20 over 6 to 12 months, your doctor may consider a kidney transplant.
Blood urea nitrogen (BUN)
A BUN is a blood test that measures the amount of urea nitrogen in your blood. Urea nitrogen is a waste product your body makes from the breakdown of protein in the foods you eat. Healthy kidneys filter urea nitrogen out of your blood, which leaves your body through your urine. This process helps keep your BUN level within a normal range.
A normal BUN level depends on your age and other health conditions, but usually ranges from 7 to 20. If your BUN level is higher than normal, this may be a sign that your kidneys aren't working well. As kidney disease progresses, your BUN level goes up.
Other tests
Chronic kidney disease.
The Chronic Kidney Disease (CKD) Initiative provides public health strategies for promoting kidney health.
For Everyone
Public health.

An official website of the United States government
The .gov means it’s official. Federal government websites often end in .gov or .mil. Before sharing sensitive information, make sure you’re on a federal government site.
The site is secure. The https:// ensures that you are connecting to the official website and that any information you provide is encrypted and transmitted securely.
- Publications
- Account settings
Preview improvements coming to the PMC website in October 2024. Learn More or Try it out now .
- Advanced Search
- Journal List
- J Int Soc Sports Nutr
Common questions and misconceptions about creatine supplementation: what does the scientific evidence really show?
Jose antonio.
1 Department of Health and Human Performance, Nova Southeastern University, Davie, Florida USA
Darren G. Candow
2 Faculty of Kinesiology and Health Studies, University of Regina, Regina, Canada
Scott C. Forbes
3 Department of Physical Education, Faculty of Education, Brandon University, Brandon, MB Canada
Bruno Gualano
4 Applied Physiology & Nutrition Research Group; School of Medicine, FMUSP, University of Sao Paulo, Sao Paulo, SP Brazil
Andrew R. Jagim
5 Sports Medicine Department, Mayo Clinic Health System, La Crosse, WI USA
Richard B. Kreider
6 Exercise & Sport Nutrition Lab, Human Clinical Research Facility, Department of Health & Kinesiology, Texas A&M University, College Station, USA
Eric S. Rawson
7 Department of Health, Nutrition, and Exercise Science, Messiah University, Mechanicsburg, PA USA
Abbie E. Smith-Ryan
8 Department of Exercise and Sport Science, University of North Carolina, Chapel Hill, NC USA
Trisha A. VanDusseldorp
9 Department of Exercise Science and Sport Management, Kennesaw State University, Kennesaw, GA USA
Darryn S. Willoughby
10 School of Exercise and Sport Science, University of Mary Hardin-Baylor, Belton, TX USA
Tim N. Ziegenfuss
11 The Center for Applied Health Sciences, Canfield, Ohio USA
Associated Data
Not applicable.
Supplementing with creatine is very popular amongst athletes and exercising individuals for improving muscle mass, performance and recovery. Accumulating evidence also suggests that creatine supplementation produces a variety of beneficial effects in older and patient populations. Furthermore, evidence-based research shows that creatine supplementation is relatively well tolerated, especially at recommended dosages (i.e. 3-5 g/day or 0.1 g/kg of body mass/day). Although there are over 500 peer-refereed publications involving creatine supplementation, it is somewhat surprising that questions regarding the efficacy and safety of creatine still remain. These include, but are not limited to: 1. Does creatine lead to water retention? 2. Is creatine an anabolic steroid? 3. Does creatine cause kidney damage/renal dysfunction? 4. Does creatine cause hair loss / baldness? 5. Does creatine lead to dehydration and muscle cramping? 6. Is creatine harmful for children and adolescents? 7. Does creatine increase fat mass? 8. Is a creatine ‘loading-phase’ required? 9. Is creatine beneficial for older adults? 10. Is creatine only useful for resistance / power type activities? 11. Is creatine only effective for males? 12. Are other forms of creatine similar or superior to monohydrate and is creatine stable in solutions/beverages? To answer these questions, an internationally renowned team of research experts was formed to perform an evidence-based scientific evaluation of the literature regarding creatine supplementation.
Introduction
Creatine (methylguanidine-acetic acid) is endogenously formed from reactions involving the amino acids arginine, glycine and methionine in the kidneys and liver [ 1 ]. Exogenously, creatine is primarily consumed from meat and/or as a dietary supplement. According to PubMed (archive of biomedical and life sciences journal literature at the U.S. National Institutes of Health’s National Library of Medicine) there are over 500 peer-refereed publications involving various aspects of creatine supplementation. Based on the enormous popularity of creatine supplementation, the International Society of Sports Nutrition (ISSN) published an updated position stand in 2017 on the safety and efficacy of creatine supplementation in exercise, sport, and medicine [ 2 ]. This comprehensive paper provided an evidence-based review of the literature examining the effects of creatine supplementation on performance, recovery, injury prevention, exercise tolerance and rehabilitation, neuroprotection, aging, clinical and disease state populations, and pregnancy. Importantly, the safety profile of creatine was also reviewed. As of September 1, 2020, the paper has been viewed 179,000 times and cited 100 times (according to Web of Science). Furthermore, Altmetric data indicates that the paper has been mentioned in 19 news outlets, 4 blogs, 492 tweets, 54 Facebook pages, and been uploaded 69 times in video posts. Instagram stories and posts are not included as Altmetric data.
Despite the widespread outreach of the 2017 ISSN position stand paper [ 2 ], along with other evidence-based review/meta-analysis papers involving various aspects of creatine supplementation published after the 2015 Creatine in Health, Sport and Medicine Conference in Germany [ 3 – 34 ], questions and misconceptions involving creatine supplementation still remain. These include, but are not limited to: 1. Does creatine supplementation lead to water retention? 2. Is creatine is an anabolic steroid? 3. Does creatine supplementation cause kidney damage / renal dysfunction? 4. Does creatine supplementation cause hair loss / baldness? 5. Does creatine supplementation lead to dehydration and muscle cramping? 6. Is creatine supplementation harmful for children and adolescents? 7. Does creatine supplementation increase body fat? 8. Is a creatine supplementation ‘loading-phase’ required? 9. Is creatine supplementation beneficial for older adults? 10. Is creatine supplementation only useful for resistance/power type activities? 11. Is creatine supplementation only effective for males? 12. Are other forms of creatine similar or superior to monohydrate? Is creatine stable in solutions/beverages? To address these questions, an internationally renowned team of research experts, who have collectively published over 200 peer-refereed articles involving creatine supplementation, was formed to perform an evidence-based scientific evaluation of the literature. Each question was answered by one researcher, chosen according to her/his expertise on the topic. Then, the final version of this manuscript was reviewed and approved by all authors, therefore reflecting the group opinion.
Does creatine lead to water retention?
The purported myth of creatine supplementation increasing body water (TBW) is likely due to early research which showed that creatine supplementation at 20 g/day for six days was associated with water retention [ 35 ]. It does appear that the most common adverse effect of creatine supplementation is water retention in the early stages (first several days) [ 36 ]. For example, studies have shown that three days of creatine supplementation increased TBW and extracellular body water (ECW) [ 37 ] and intracellular water (ICW) [ 38 ]. Unfortunately, based on these short-term responses, this notion that creatine increases water retention over the long-term has been widely accepted [ 39 ].
Creatine is an osmotically active substance. Thus, an increase in the body's creatine content could theoretically result in increased water retention. Creatine is taken up into muscle from circulation by a sodium-dependent creatine transporter [ 1 ]. Since the transport involves sodium, water will also be taken up into muscle to help maintain intracellular osmolality. However, considering the activity of the sodium-potassium pumps, it is not likely that intracellular sodium concentration is dramatically affected by creatine supplementation [ 39 ].
A number of exercise training studies (e.g., 5-10 weeks) incorporating creatine supplementation have shown no increases in total body water (TBW). For example, resistance-trained males who received creatine at a dose of 0.3 g/kg lean body mass/day for 7 days (approximately 20 g/day) followed by 4 weeks at 0.075 g/kg lean body mass/day for 28 days (approximately 5 g/day) experienced no significant change in ICW, ECW, or TBW [ 40 ]. Furthermore, resistance-trained males who consumed creatine supplementation (20 g/day for seven days followed by 5 g/day for 21 days) had no significant increase in ICW, ECW, or TBW [ 41 ]. Similarly, males and females ingesting creatine (0.03 g/kg/day for six weeks) experienced no significant increase in TBW [ 42 ]. Six weeks of creatine supplementation in non-resistance-trained males at a dosage of 0.3 g/kg lean body mass for five days followed by 0.075 g/kg lean body mass for 42 days produced no significant changes in TBW [ 43 ]. In contrast, when assessing TBW, ICW, and ECW content before and after 28 days of creatine supplementation in healthy males and females (n = 32), Powers et al. [ 44 ] showed that creatine supplementation was effective at increasing muscle creatine content which was associated with an increase in body mass and TBW but did not alter ICW or ECW volumes. In a recent study examining the effects of creatine supplementation combined with resistance exercise for 8 weeks, Ribeiro et al. [ 45 ] found a significant increase in TBW (7.0%) and ICW (9.2%) volume compared to placebo (TBW: 1.7%; ICW: 1.6%), with both groups similarly increasing ECW (CR: 1.2% vs. Placebo = 0.6%). Importantly, the ratio of skeletal muscle mass to ICW remained similar in both groups. It is important to highlight that the ICW is an important cellular signal for protein synthesis and thus drives an increase in muscle mass over time [ 46 ].
In summary, while there is some evidence to suggest that creatine supplementation increases water retention, primarily attributed to increases in intracellular volume, over the short term, there are several other studies suggesting it does not alter total body water (intra or extracellular) relative to muscle mass over longer periods of time. As a result, creatine supplementation may not lead to water retention.
Is creatine an anabolic steroid?
Anabolic steroids are a synthetic version of testosterone, an androgenic hormone which is also produced endogenously within both males and females, and is used in conjunction with resistance training with the intent of enhancing muscle mass and strength due to increases in muscle protein synthesis [ 47 ]. This increase in MPS is due to testosterone’s ability to enter the muscle cell, bind with the intracellular androgen receptor, and increase the expression of various muscle-specific genes [ 48 ]. Creatine is converted to phosphocreatine (PCr), regulated by the enzyme creatine kinase (CK) in muscle and used to create intracellular adenosine triphosphate (ATP) production [ 1 ]. Creatine supplementation, however, can increase the capacity of ATP and energy produced during heavy anaerobically-related exercise, thereby possibly increasing muscle power, repetitions and exercise volume which can subsequently contribute to muscle performance and hypertrophy over the course of a training period [ 2 ].
While the physiological and performance outcomes of anabolic steroids and creatine can be similar, their mechanisms of action and legal categorization are not. Anabolic steroids are drugs, with a different chemical structure than creatine, and are Class C, Schedule III controlled substances regulated by the Food and Drug Administration (FDA) and subject to the regulatory control provisions of the Controlled Substances Act (CSA) set forth by the Drug Enforcement Association (DEA). Creatine, on the other hand, like many other dietary supplements fits well within the confines of The Dietary Supplement Health and Education Act of 1994 ("DSHEA"), which is a statute of United States Federal legislation which defines and regulates dietary supplements by the Federal Drug Administration (FDA) for Good Manufacturing Practices (GMP). It is illegal to possess and administer anabolic steroids without a physician’s prescription. However, there are no legal ramifications for the possession or ingestion of creatine.
In summary, because creatine has a completely different chemical structure, it is not an anabolic steroid.
Does creatine cause kidney damage/renal dysfunction?
Questions and concerns involving creatine supplementation and kidney damage/renal dysfunction are common. In terms of pervasive misinformation in the sport nutrition arena, the notion that creatine supplementation leads to kidney damage/renal dysfunction is perhaps second only to the myth that protein supplementation and high habitual protein intake causes kidney damage. Today, after > 20 years of research which demonstrates no adverse effects from recommended dosages of creatine supplements on kidney health, unfortunately, this concern persists. While the origin is unknown, the connection between creatine supplementation and kidney damage/renal dysfunction could be traced back to two things: a poor understanding of creatine and creatinine metabolism and a case study published in 1998.
In skeletal muscle, both creatine and PCr are degraded non-enzymatically to creatinine, which is exported to the blood and excreted in the urine [ 1 ]. Healthy kidneys filter creatinine, which would otherwise increase in the blood. Therefore, blood creatinine levels can be used as a proxy marker of kidney function. However, the amount of creatinine in the blood is related to muscle mass (i.e. males have higher blood creatinine than females) and both dietary creatine and creatinine intake [ 35 ]. Both blood and urinary creatinine may be increased by ingestion of creatine supplementation and creatine containing foods, such as meat. Creatine is normally not present in urine, but can reach very high levels (>10 g/day) during creatine supplementation [ 49 ]. There appears to be an unsubstantiated perspective that if the kidneys are “forced” to excrete higher than normal levels of creatine or creatinine, some sort of kidney “overload” will take place, causing kidney damage and/or renal dysfunction. In reality, transient increases in blood or urinary creatine or creatinine due to creatine supplementation are unlikely to reflect a decrease in kidney function. Additionally, one must exercise caution when using blood creatinine and estimated creatinine clearance/glomerular filtration rate in individuals who consume high meat intake or supplement with creatine. In a review of creatine supplementation studies, Persky and Rawson [ 50 ] found no increase in serum creatinine in 12 studies, 8 studies showed an increase that remained within the normal range, and only 2 studies showed an increase above normal limits (although not different from the control group in one study).
In 1998, a case study of a young male with focal segmental glomerulosclerosis and relapsing nephrotic syndrome was reported [ 51 ]. The young male, who had kidney disease for 8 years and was treated with cyclosporine (i.e., immunosuppressant) for 5 years, had recently begun ingesting creatine supplementation (15 g/day for 7 days; followed by 2 g/day for 7 weeks). Based on increased blood levels of creatinine and subsequent estimate of calculated creatinine clearance, his kidney health was presumed to be deteriorating, although he was otherwise in good health. The patient was encouraged to discontinue creatine supplementation. At this time, it was already known that blood and urine creatinine levels can increase following ingestion of creatine containing food products, including creatine supplements [ 35 ]. This was ignored by the authors of this case study, as was the inclusion of two investigations which demonstrated that creatine supplementation did not negatively impact renal function [ 52 , 53 ]. The dosage of creatine during the maintenance phase, which was also ignored, was only slightly higher than the daily creatine intake of a typical omnivore’s dietary intake, or in terms of food, a large hamburger or steak per day (meat contains about 0.7 g of creatine / 6 oz. serving; see [ 54 ]). In response to this case study, two separate teams of experts in creatine metabolism wrote letters to the editor of Lancet [ 53 , 55 ]. However, the notion that creatine supplementation leads to kidney damage and/or renal dysfunction gained traction and momentum.
Since this case study was reported in 1998, experimental and controlled research trials investigating the effects of creatine supplementation on kidney/renal function has substantially increased [ 50 , 56 – 58 ]. Overall, in healthy individuals, there appears to be no adverse effects from consuming recommended doses of creatine supplements on kidney/renal function [ 50 , 56 – 58 ]. Interestingly, Gualano et al. [ 58 ] reviewed a small number of case studies which reported renal dysfunction in individuals who were supplementing with creatine. Similar to the case report by Pritchard and Kalra [ 51 ], these additional case reports were confounded by medications, pre-existing kidney disease, concomitant supplement ingestion, inappropriate creatine dosages (e.g., 100 X recommended dose), and anabolic androgenic steroid use.
It is prudent to be cautious when ingesting any dietary supplement or medication. Survey data indicates that creatine supplementation usage ranges between 8-74% in athletes and other exercising individuals (reviewed in Rawson et al. [ 59 ]). Even with a low estimate of 8% of exercising individuals using creatine supplements, this indicates thousands of exposures across several decades. If the link between creatine supplementation and kidney health was valid, there would be an expected increase in kidney damage / renal dysfunction in low risk (i.e. young, physically fit, healthy) individuals since 1992 after Harris et al. published their seminal work [ 60 ]. After nearly 30 years of post-marketing surveillance, thousands of exposures, and multiple clinical trials, no such evidence exists.
In summary, experimental and controlled research indicates that creatine supplementation, when ingested at recommended dosages, does not result in kidney damage and/or renal dysfunction in healthy individuals.
Does creatine cause hair loss / baldness?
The vast majority of speculation regarding the relationship between creatine supplementation and hair loss/baldness stems from a single study by van der Merwe et al. [ 61 ] where college-aged male rugby players who supplemented with creatine (25 g/day for 7 days, followed by 5 g/day thereafter for an additional 14 days) experienced an increase in serum dihydrotestosterone (DHT) concentrations over time. Specifically, DHT increased by 56% after the seven-day loading period, and remained 40% above baseline values after the 14-day maintenance period. These results were statistically significant compared to when the subjects consumed a placebo (50 g of glucose per day for 7 days, followed by 30 g/day for 14 days thereafter). Given that changes in these hormones, particularly DHT, have been linked to some (but not all) occurrences of hair loss/baldness [ 62 ], the theory that creatine supplementation leads to hair loss / baldness gained some momentum and this potential link continues to be a common question / myth today. It is important to note that the results of van der Merwe et al. [ 61 ] have not been replicated, and that intense resistance exercise itself can cause increases in these androgenic hormones.
DHT is a metabolite of testosterone, formed when the enzyme 5-alpha-reductase converts free testosterone to DHT [ 63 ]. In males, DHT can bind to androgen receptors in susceptible hair follicles and cause them to shrink, ultimately leading to hair loss [ 64 ]. However, in the van der Merwe et al. [ 61 ] study, no increase in total testosterone was found in the 16 males who completed the study. Free testosterone was not measured. Moreover, the increase in DHT and the DHT: testosterone ratio remained well within normal clinical limits. Furthermore, baseline (prior to supplementation), DHT was 23% lower in the creatine group (0.98 nmol/L) compared to the placebo group (1.26 nmol/L). Thus the small increase in DHT in the creatine group (+ 0.55 nmol/L after 7 days of supplementation and + 0.40 nmol/L after 21 days of supplementation), in combination with a small decrease in the placebo DHT response (-0.17 nmol/L after 7 days of supplementation and -0.20 nmol/L after 21 days of supplementation) explains the “statistically significant” increase in DHT noted by van der Merwe et al. [ 61 ]. While it is possible that creatine supplementation upregulated 5-alpha-reductase activity in these males (potentially leading to increased formation of DHT), no study has reported hair loss/baldness in humans.
To date, 12 other studies have investigated the effects of creatine supplementation (i.e. doses ranging from 3-25 g/day for 6 days to 12 weeks) on testosterone. Two studies reported small, physiologically insignificant increases in total testosterone after six and seven days of supplementation [ 65 , 66 ], while the remaining ten studies reported no change in testosterone concentrations. In five of these studies [ 67 – 71 ], free testosterone, which the body uses to produce DHT, was also measured and no increases were found.
In summary, the current body of evidence does not indicate that creatine supplementation increases total testosterone, free testosterone, DHT or causes hair loss/baldness.
Does creatine lead to dehydration and muscle cramping?
Speculation exists that creatine supplementation causes dehydration and muscle cramping [ 72 , 73 ]. In the early 2000’s, with limited data and based primarily on speculation, the American College of Sports Medicine (ACSM) recommended that individuals controlling their weight and exercising intensely or in hot environments should avoid the use of creatine supplementation [ 74 ]. The physiological rationale suggesting that creatine supplementation may cause dehydration and muscle cramping is based on the premise that creatine is an osmotically active substance found primarily in skeletal muscle and may alter whole-body fluid distribution by preferentially increasing intracellular water uptake and retention, particularly over the short-term [ 38 , 75 ]. In situations of body water loss, such as severe sweating from exercise and/or increased environmental temperature, the bound intracellular fluid, in theory, may be detrimental to thermal regulation and lead to extracellular dehydration, electrolyte imbalance and muscle cramping or other heat-related musculoskeletal issues [ 44 ]. The initial loading phase of creatine supplementation (i.e. 20 g/day for 5-7 days) typically results in a 1-3 kg increase in body mass, mostly attributable to net body water retention [ 75 , 76 ]. Some anecdotal evidence indicates that creatine users perceive supplementation to result in some adverse effects [ 77 ]. For example, in a survey involving 219 athletes, 90 participants reported using creatine with 34 of them (38%) reporting perceived negative effects such as cramping (27%) [ 77 ]. Similarly, in National Collegiate Athletic Association (NCAA) Division 1 baseball and football players (N=52) using creatine, 25% reported incidences of muscle cramping and 13.5% reported symptoms of dehydration. Importantly, these studies failed to control for the use of other supplements and the dosage of creatine ingested. Greenwood et al. [ 77 ] noted that 91% of participants exceeded the recommended creatine maintenance dose of 5 g/day. However, these self-report surveys are in contradiction to experimental and clinical evidence. Greenwood et al. [ 78 ] monitored injury rates in Division IA NCAA collegiate football players (N=72; age: 19.7 ± 1.0 yrs) where environmental conditions were hot (27.3 ± 10.9 0 C) and humid (54.2 ± 9.7%). Participants chose to receive either creatine (n = 38: 0.3 g/kg/day for 5 days; followed by 0.03 g/kg/day for 115 days) or a sport drink placebo (n = 34) throughout the football season. Injuries treated by the athletic training staff were monitored. Creatine users had significantly less cramping (p = 0.021), heat illnesses and dehydration (p = 0.043), muscle tightness (p = 0.020), muscle strains (p = 0.021), and total injuries (p < 0.001) compared to non-users. Non-contact joint injuries, contact injuries, illnesses, missed practices due to injuries, and players lost for the season were not different between groups. In a clinical setting, haemodialysis patients (n = 10) who frequently reported muscle cramping were provided creatine (12 g) 5 minutes prior to haemodialysis [ 79 ]. Creatine supplementation reduced the frequency of symptomatic muscle cramping by 60% [ 79 ]. These beneficial effects from creatine may be explained by fluid distribution and electrolyte imbalances, as previously discussed.
In summary, experimental and clinical research does not validate the notion that creatine supplementation causes dehydration and muscle cramping.
Is creatine harmful for children and adolescents?
Concerns regarding the safety of creatine supplementation in children and adolescents (< 19 yrs) continues to be highly prevalent. The overwhelming majority of evidence in adult populations indicates that creatine supplementation, both short- and longer-term, is safe and generally well tolerated [ 2 ]. However, the question of whether or not this holds true for children and adolescents is relatively unclear. The physiological rationale supporting the potential ergogenic benefits of creatine supplementation in children and adolescents was first postulated by Unnithan and colleagues in 2001 [ 80 ]; which established a strong basis for future applications of creatine for younger athletes. More recently, in a comprehensive review examining the safety of creatine supplementation in adolescents, Jagim et al. [ 16 ] summarized several studies that examined the efficacy of creatine supplementation among various adolescent athlete populations and found no evidence of adverse effects. However, it is important to note that none of the performance-focused studies included in the Jagim et al. [ 16 ] review provided data examining specific markers of clinical health and whether or not they were impacted by the supplementation protocols.
From a clinical perspective, creatine supplementation has been found to potentially offer health benefits with minimal adverse effects in younger populations. Hayashi et al. [ 81 ] found improvements in pediatric patients with systemic lupus erythematosus and reported no adverse changes in laboratory parameters of hematology, kidney function, liver function or inflammatory markers after 12 weeks of creatine supplementation. Tarnopolsky et al. [ 82 ] reported significant improvements in fat-free mass and hand grip strength in 30 pediatric patients with Duchenne muscular dystrophy following 4 months of creatine supplementation. Importantly, the creatine supplementation protocol appeared to be well tolerated and did not adversely affect laboratory markers of kidney function, oxidative stress, and bone health [ 81 – 83 ]. In addition, Sakellaris et al. [ 83 ] reported significant improvements in traumatic brain injury-related outcomes in children and adolescents who received oral creatine supplementation (0.4 g/kg/day) for 6 months. These neurological benefits may have potential applications for young athletes participating in collision sports, which pose underlying risks of concussions or sub-concussive impacts. Further, several of these clinical trials implemented strict clinical surveillance measures, including continual monitoring of laboratory markers of kidney health, inflammation, and liver function; none of which were negatively impacted by the respective creatine supplementation interventions. These findings support the hypothesis of creatine supplementation likely being safe for children and adolescents. However, perhaps the strongest supporting evidence for the safety of creatine is the recent classification of creatine as generally recognized as safe (GRAS) by the United States Food and Drug Administration (FDA) in late 2020 ( https://www.fda.gov/media/143525/download ). Ultimately, this classification indicates that the currently available scientific data pertaining to the safety of creatine, is sufficient and has been agreed upon by a consensus of qualified experts, thereby determining creatine to be safe under the conditions of its intended use ( https://www.fda.gov/media/143525/download ). Even though infants and young children are excluded from GRAS, this would still apply to older children and adolescent populations.
The majority of dietary supplement survey data indicates that a relatively high percentage of youth and adolescent athletes are currently or have previously supplemented with creatine. For example, Kayton et al. [ 84 ] found that in a sample of 270 high school boys and girls, 21% of boys and 3% of girls reported supplementing with creatine. Furthermore, in a sample of elite Olympic level sample of young German athletes (14-18 yrs), 12% of those surveyed reported supplementing with creatine [ 85 ]. Therefore, these trends warrant additional research to determine with greater certainly whether creatine supplementation, both acute and longer-term, is safe for children and adolescents.
In summary, based on the limited evidence, creatine supplementation appears safe and potentially beneficial for children and adolescents.
Does creatine increase fat mass?
The theory that creatine supplementation increases fat mass is a concern amongst exercising individuals, possibly because some experience a gain in body mass from creatine supplementation. However, randomized controlled trials (one week to two years in duration) do not validate this claim. Acute creatine supplementation (7 days) had no effect on fat mass in young and older adults; however, fat-free mass was increased [ 86 , 87 ]. Furthermore, three weeks of creatine supplementation had no effect on body composition in swimmers [ 88 ]. The addition of creatine to high-intensity interval training had no effect on body composition in recreationally active females [ 89 ]. In addition, the effects of creatine supplementation during resistance training overreaching had no effect on fat mass [ 70 ]. Moreover, in a group of healthy recreational male bodybuilders, 5 g/day of creatine consumed either pre- or post-training had no effect on fat mass [ 90 ]. In other short-terms studies lasting 6-8 weeks, there were no changes in fat mass from creatine supplementation. Becque et al. [ 91 ] found no changes in fat mass after six weeks of supplementation plus resistance training. In another 6-week investigation, no significant differences in fat mass or percentage body fat were observed after creatine supplementation [ 42 ]. Furthermore, creatine supplementation during an 8-week rugby union football season also had no effect on fat mass [ 92 ].
One might suggest that eight weeks or less of creatine supplementation is insufficient to arrive at a definitive conclusion regarding creatine’s effect on fat mass. Nonetheless, there are several investigations that have used much longer treatment periods. For example, healthy resistance-trained males were randomly assigned in a double-blind fashion to supplement with creatine (i.e., 20 g/day for 1 week followed by 5 g/day for 11 weeks) or placebo [ 93 ]. Lean body mass and muscle fiber size increased; percent body fat and fat mass were unaffected over the 12-week training period [ 93 ]. In older males (~70 yrs), 12 weeks of creatine supplementation during resistance training had no effect (compared to placebo) on fat mass [ 94 ]. Furthermore, Gualano et al. assessed the effects of creatine supplementation (24 weeks), with and without resistance training, in older females. Results showed no effect from creatine on fat mass [ 95 ]. Candow et al. [ 96 ] examined the effects of creatine supplementation in older adults (50-71 years) over a 32-week treatment period. Study participants were randomized to supplement with creatine or placebo before or after resistance training (3 days per week). There was an increase over time for lean tissue and strength with a decrease in fat mass. From a clinical perspective, children with acute lymphoblastic leukemia who supplemented with creatine (0.1 g/kg/day) for two sequential periods of 16 weeks experienced a significant reduction in fat mass. In contrast, the children who did not consume creatine gained fat mass [ 97 ]. In two studies involving postmenopausal women, Lobo et al. [ 98 ] found no change in absolute or relative body fat from one-year of low-dose creatine supplementation. Furthermore, two years of creatine supplementation also had no effect on fat mass [ 99 ].
Recently, Forbes et al. [ 100 ] conducted a systematic review and meta-analysis on randomized controlled trials involving creatine supplementation in conjunction with resistance training on fat mass in older adults (≥ 50 yrs). Nineteen studies with a total of 609 participants were included. Participants supplementing with creatine had a greater reduction in body fat percentage. There was no significant difference in absolute fat mass loss; however, the creatine group lost ~0.5 kg more fat mass compared to those on placebo.
In summary, creatine supplementation does not increase fat mass across a variety of populations.
Is a creatine ‘loading-phase’ required?
Pioneering research in the early 1900’s using animal models showed that creatine supplementation could augment creatine content by 70% [ 101 , 102 ]. Decades later, Harris et al. [ 60 ] published a seminal paper which showed that ‘loading’ with creatine increased skeletal muscle creatine stores, as evaluated from muscle biopsies collected from the vastus lateralis in young, healthy human participants. This research sparked incredible interest in studying creatine supplementation strategies that would increase intramuscular creatine content, helping shape current recommendations.
Creatine ‘loading’ is defined as supplementing with oral creatine for 5–7 days with a dosage of 20–25 g/day, often divided into smaller doses throughout the day (e.g., four to five, 5 g servings/day). Creatine ‘loading’ may also be prescribed relative to body mass, for example, 0.3 g/kg/d for 5-7 days (i.e., 21 g/day for a 70 kg individual). The ‘loading’ phase of creatine supplementation is followed by a daily ‘maintenance’ phase often ranging from daily 3–5 g servings/day (Figure (Figure1, 1 , side A). In addition to the seminal work of Harris et al. [ 60 ], several other investigations have demonstrated increased intramuscular creatine stores in humans from the creatine ‘loading’ phase [ 35 , 103 , 104 ]. A common misconception regarding creatine supplementation is that individuals must ‘load’ with creatine to increase intramuscular creatine stores and subsequently experience the purported ergogenic benefits of creatine supplementation. However, lower daily creatine supplementation dosing strategies (i.e., 3-5 g/day) are well established throughout the scientific literature for increasing intramuscular creatine stores leading to greater improvements in muscle mass, performance and recovery compared to placebo [ 2 ]. While effective, these non-loading creatine supplementation dosing strategies (Figure (Figure1, 1 , side B) delay maximum intramuscular creatine storage. For example, in the classic ‘loading’ vs. daily ‘maintenance’ dose comparison study by Hultman et al. [ 35 ], creatine accumulation in muscle was similar (~ 20% increase) after participants consumed 3 g/day for 28 days or 20 g/day for 6 days [ 35 ]. Thus, it is currently recommended that individuals consume ~3-5 g/day of creatine for a minimum of 4 weeks in order to experience similar skeletal muscle saturation levels. Determination of which creatine supplementation strategy is preferred may depend on the goal of the individual. For instance, if an athlete is hoping to maximize the ergogenic potential of creatine supplementation in a very short period of time (< 30 days), adopting the creatine ‘loading’ strategy may be advised. However, if an athlete or exercising individual is planning to ingest creatine over an extended period of time (> 30 days), or if avoiding potential weight gain which can sometimes occur during creatine ‘loading’, the creatine ‘maintenance’ strategy would be a viable option. Athletes who are carrying out a creatine loading phase (i.e., 20 g/day) should emphasize the smaller dosing strategies (e.g. less than or equal to 10 gram servings) throughout the day, as dosages of greater than 10 grams may potentially lead to gastrointestinal distress (i.e., diarrhea) [ 105 ].

Creatine supplementation strategies.
In summary, accumulating evidence indicates that you do not have to ‘load’ creatine. Lower, daily dosages of creatine supplementation (i.e. 3-5 g/day) are effective for increasing intramuscular creatine stores, muscle accretion and muscle performance/recovery.
Is creatine beneficial for older adults?
There has been an increasing number of studies showing that creatine supplementation plays a therapeutic role in a variety of clinical conditions (see Gualano et al. [ 106 ] for a comprehensive review on this topic).
Perhaps one of the most promising conditions that could benefit from creatine supplementation is age-related sarcopenia. Sarcopenia is defined as a progressive and generalized skeletal muscle condition (i.e. decrease in muscle mass, strength, and functionality) that is associated with increased likelihood of adverse outcomes including falls, fractures, physical disability and mortality [ 107 ]. While resistance training is considered cornerstone in the treatment of sarcopenia [ 108 ], accumulating evidence indicates that creatine supplementation may enhance the anabolic environment produced by resistance training, subsequently mitigating indices of sarcopenia [ 9 , 10 , 19 , 27 ].
Creatine supplementation can increase functionality (e.g., strength, activities of daily living, delay fatigue) and muscle mass in older adults [ 9 , 10 , 19 , 87 , 95 , 109 , 110 ]. However, the literature indicates that creatine alone (that is, without a concomitant resistance training program) is unlikely to result in substantial gains in muscle strength and functional performance [ 95 , 111 – 113 ], although it does improve some parameters of muscle fatigue [ 114 – 116 ]. Likewise, most studies failed to show a beneficial effect of chronic creatine supplementation alone (≥ 30 days) on lean mass [ 98 , 99 , 113 , 114 ]. For instance, we recently showed that creatine supplementation was not able to increase lean mass in postmenopausal women who supplemented with creatine (3 g/day) for 2 years, suggesting that creatine supplementation without exercise may be ineffective to prevent sarcopenia [ 99 ]. It is likely that increases in lean mass occasionally attributed to creatine supplementation in short-term studies (e.g., 7 days) are explained by increased body water, since creatine is osmotically active and it can sometimes induce water retention.
Conversely, substantial evidence indicates that creatine supplementation is capable of augmenting the hypertrophic response to resistance training in young adults [ 117 ], which is extended to older adults, as confirmed by three systematic reviews and meta-analyses [ 19 , 118 , 119 ]. The fact that creatine is more effective when combined with a training stimulus suggests that the main mechanistic action of creatine is its ability to enhance training volume and/or intensity, which may influence muscle protein kinetics, growth factors, satellite cells, inflammation and/or oxidative stress [ 9 , 10 , 19 ], ultimately resulting in greater skeletal muscle adaptations.
Regarding aging bone, emerging research over the past decade has shown some benefits from creatine supplementation. For example, healthy older males (> 50 yrs) who supplemented with creatine and performed whole-body resistance training for 10-12 weeks experienced an increase in upper limb bone mineral content [ 120 ] and a reduction in bone resorption compared to placebo [ 121 ]. More recently, Chilibeck et al. [ 122 ] showed that 52 weeks of creatine supplementation and supervised whole-body resistance training attenuated the rate of bone mineral loss in the hip region compared to placebo in postmenopausal females. However, a 2 year creatine supplementation protocol was infective for improving bone mass or bone geometry in post-menopausal women, again suggesting that creatine should be combined with resistance-type exercise to produce beneficial bone adaptations [ 99 ].
From a clinical and healthy aging perspective, it is recommended that creatine supplementation be combined with resistance training to produce the greatest adaptations in older adults. Future clinical trials involving frail populations with long-term follow-up(s) and larger samples are needed. The therapeutic potential of creatine supplementation for cachexia, myopathies, post-surgery rehabilitation, bed rest, other muscle/bone wasting condition/diseases and brain health warrants further investigation.
In summary, there is growing body of evidence showing that creatine supplementation, particularly when combined with exercise, provides musculoskeletal and performance benefits in older adults.
Is creatine only useful for resistance / power type activities?
Although creatine supplementation has been theorized to primarily benefit athletes involved in high-intensity intermittent resistance/power type activities, there is a growing body of evidence suggesting that creatine supplementation may also provide beneficial effects for other activities. For example, creatine supplementation with carbohydrate [ 123 ] or carbohydrate and protein [ 124 ] has been reported to promote greater muscle glycogen storage than carbohydrate supplementation alone. Since glycogen replenishment is important for promoting recovery and preventing overtraining during intensified training periods [ 2 , 125 ], creatine supplementation may help athletes who deplete large amounts of glycogen during training and/or performance (i.e., sporting events) to maintain optimal glycogen levels. Second, there is evidence that creatine supplementation may reduce muscle damage and/or enhance recovery from intense exercise. For example, Cooke and colleagues [ 126 ] reported that creatine supplementation during recovery from exercise-induced muscle damage promoted less muscle enzyme efflux and better maintenance of isokinetic muscle performance. Moreover, there is evidence that individuals supplementing their diet with creatine experienced less muscle damage, inflammation, and muscle soreness in response to running 30-km [ 127 ] as well as during 4-weeks of intensified training [ 70 ]. Consequently, creatine supplementation may help athletes recover from intense exercise and/or tolerate intensified periods of training to a greater degree. Third, there is evidence that athletes who supplement with creatine during training experience fewer musculoskeletal injuries, accelerated recovery time from injury [ 78 , 128 ] and less muscle atrophy after immobilization [ 129 , 130 ]. Whether this is due to a greater resistance to injury and/or ability to recover from injury remains unclear. Fourth, creatine supplementation (with or without glycerol) has been reported to help athletes hyper-hydrate and thereby enhance tolerance to exercise in the heat [ 28 , 37 , 131 – 145 ]. Therefore, creatine supplementation may reduce the risk of heat related-illness when athletes train and/or compete in hot and humid environments [ 72 , 146 ]. Finally, there is evidence from animal models that creatine supplementation is neuroprotective [ 147 – 149 ] and can reduce the severity of spinal cord injury [ 150 , 151 ], cerebral ischemia [ 152 – 155 ], and concussion/traumatic brain injury [ 2 , 7 , 12 , 22 , 32 , 33 , 156 ]. This evidence was so compelling that the International Society of Sports Nutrition recommended that athletes engaged in sports that have a potential for concussion and/or spinal cord injury take creatine for its neuroprotective effects [ 2 ]. Thus, there are a number of reasons beyond the ergogenic benefit that all types of athletes may benefit.
In summary, there is a variety of athletic events, not just resistance/power activities, which may benefit from creatine supplementation.
Is creatine only effective for males?
Creatine kinetics may vary between healthy males and females [ 157 ]. Females may have higher intramuscular creatine concentrations [ 158 ] possibly due to lower skeletal muscle mass [ 159 ]. Potentially, the higher resting intramuscular creatine concentration in females (based on the upper limit of intramuscular creatine storage) may help explain some research showing diminished responsiveness and/or performance effects on females [ 160 , 161 ].
As a result of hormone-driven changes in endogenous creatine synthesis, creatine transport, and creatine kinase (CK) kinetics, creatine bioavailability throughout various stages of female reproduction is altered, highlighting the potential positive implications for creatine supplementation in females [ 29 ]. The implications of hormone-related changes in creatine kinetics has been largely overlooked in performance-based studies [ 29 ]. Specifically, creatine supplementation may be of particular importance during menses, pregnancy, post-partum, perimenopause and postmenopause. Creatine kinase, as well as enzymes associated with creatine synthesis, are influenced by estrogen and progesterone [ 1 ]. Creatine kinase levels are significantly elevated during menstruation [ 162 ], with CK levels decreasing throughout the menstrual cycle, pregnancy, and with age. The lowest range of CK values have been reported during early pregnancy (20 weeks or less), equating to about half the concentration found at peak levels (teenage girls) [ 162 , 163 ].
Maternal creatine supplementation during pregnancy in pre-clinical animal studies have demonstrated a protective effect against fetal death and organ damage associated with intrapartum hypoxia [ 164 , 165 ]. Reduced creatine levels in late pregnancy have also been associated with low fetal growth [ 165 ]. There is additional data that metabolic demand from the placenta during gestation further lowers the creatine pool of the mother [ 166 ], which may be associated with low birth weight and pre-term birth. Creatine supplementation during pregnancy has been shown to enhance neuronal cell uptake of creatine and support mitochondrial integrity in animal offspring, thereby reducing brain injury induced by intrapartum asphyxia [ 167 , 168 ]. Although there are no human studies evaluating the effects of creatine supplementation during pregnancy, creatine could provide a safe, low-cost nutritional interventional for reducing intra- and post-partum complications associated with cellular energy depletion [ 169 ]. This may be more important if the female is vegetarian, or unable to consume meat due to nausea or taste preferences (i.e. meat contains about 0.7 g of creatine/6 oz serving [ 54 ];).
Females have been reported to have lower levels of creatine in the brain (frontal lobe) [ 170 ]. Increasing creatine concentrations in the brain as a result of supplementation, particularly in females, may support the reported benefits of reducing symptoms of depression [ 171 , 172 ] and ameliorating the effects of traumatic brain injury [ 12 , 22 ]. Depression is about 2 times higher among females throughout the reproductive years [ 173 ] and accelerates around pubertal hormonal changes [ 174 ]. Altered brain bioenergetics and mitochondrial dysfunction have been linked with depression, particularly as it relates to CK, ATP, and inorganic phosphate (P i ). Creatine supplementation has been shown to significantly augment cerebral PCr and P i [ 175 ], particularly in females. The increase in cerebral PCr from 10 g of creatine supplementation was reported to be inversely related to symptoms of depression in adolescent females resistant to selective serotonin reuptake inhibitors [ 171 ] It appears that creatine supplementation may be effective for supporting creatine kinetics, mood, and pregnancy/fetal outcomes.
There is a small body of research that has investigated the effects of creatine supplementation in younger females. For example, Vandenberghe et al. [ 176 ] showed that creatine supplementation (20 g/day for 4 days followed by 5 g/day thereafter) during 10 weeks of resistance training significantly increased intramuscular concentrations, muscle mass and strength compared to placebo in females (19-22 yrs). In elite female soccer players (22 ± 5 yrs), creatine supplementation (20 g/day for 6 days) improved sprint and agility performance compared to placebo [ 177 ]. Hamilton et al. [ 178 ] showed that creatine supplementation (25 g for 7 days) augmented upper-body exercise capacity in strength-trained females (21-33 yrs) compared to placebo (19-29 yrs). Furthermore, in college-aged females (20 yrs), creatine supplementation (0.5 g/kg of fat-free mass for 5 days) improved knee extension muscle performance compared to placebo [ 179 ]. In contrast, not all data show improved performance in females [ 89 , 160 , 161 ]. Additionally, Smith-Ryan et al. [ 180 ] reported no significant effects of creatine loading on neuromuscular properties of fatigue in young adult females. It is important to evaluate the benefit to risk ratio; as noted elsewhere in this document, there are minimal risks associated with creatine supplementation, particularly when it is evaluated against the potential benefits in females.
Accumulating research over the past decade in postmenopausal females demonstrates that creatine supplementation during a resistance training program can improve muscle mass, upper- and lower-body strength, and tasks of functionality (30-s chair stand, lying prone-to-stand test, arm curl test) (for detailed review see Candow et al. [ 9 ]). Creatine supplementation appears to be a viable option for post-menopausal females to improve muscle quality and performance. In addition to its beneficial effects on aging muscle, creatine supplementation may also have favorable effects on bone in postmenopausal females, if combined with resistance training. For example, postmenopausal females who supplemented daily with 0.1 g/kg/day of creatine during 52-weeks of supervised whole-body resistance training experienced an attenuation in the rate of bone mineral loss at the femoral neck (hip), compared to females on placebo during training [ 122 ]. Furthermore, 5 g/day of creatine supplementation during 12 weeks of resistance training in postmenopausal females resulted in a significant increase in muscle mass and upper- and lower-body strength, compared to placebo [ 181 ]. However, even without the stimulus of resistance training, there is some evidence that creatine supplementation can still be beneficial. For example, in aging females (n=10; 67 ± 6 yrs), acute creatine supplementation (0.3 g/kg/day for 7 days) significantly improved lower-extremity physical performance (sit-to-stand test) [ 110 ], and fat-free mass and upper- and lower-body strength compared to placebo [ 86 ].
In summary, there is accumulating evidence that creatine supplementation has the potential to be a multifactorial therapeutic intervention across the lifespan in females, with little to no side effects.
Are other forms of creatine similar or superior to monohydrate and is creatine stable in solutions/beverages?
Creatine monohydrate powder has been the most extensively studied and commonly used form of creatine in dietary supplements since the early 1990s [ 2 , 125 ]. Creatine monohydrate was used in early studies to assess bioavailability, determine proper dosages, and assess the impact of oral ingestion of creatine on blood creatine and intramuscular creatine stores [ 35 , 60 , 182 ]. These studies indicated that orally ingested creatine monohydrate (e.g., 3–5 g/day) increases blood concentrations of creatine for 3-4 hours after ingestion thereby facilitating the uptake of creatine into tissue through diffusion and creatine transporters [ 1 , 183 , 184 ]. Additionally, it is well established that ~99% of orally ingested creatine monohydrate is either taken up by tissue or excreted in the urine as creatine through normal digestion [ 60 , 185 , 186 ]. Short-term loading with creatine monohydrate (e.g., consuming 5 g, 4 times daily for 5-7 days) has been reported to increase intramuscular creatine stores by 20–40% and exercise performance capacity by 5–10% [ 2 , 125 ]. Creatine monohydrate supplementation during training (e.g., 5–25 g/day for 4–12 weeks) has been reported to promoted gains in muscle mass, strength, and exercise capacity [ 2 , 125 ]. Despite the known efficacy, safety, and low cost of creatine monohydrate; a number of different forms of creatine have been marketed as more effective with fewer anecdotally reported adverse effects [ 187 ]. These marketing efforts have fueled speculation that creatine monohydrate is not the most effective or safest form of creatine to consume. This notion is clearly refuted by understanding the well-known physio-chemical properties of creatine monohydrate, as well as current creatine supplementation literature.
A number of different forms of creatine (e.g., creatine salts, creatine complexed with other nutrients, creatine dipeptides, etc.) have been marketed as more effective sources of creatine than creatine monohydrate [ 187 ]. However, there are no peer-reviewed published papers showing that the ingestion of equal amounts of creatine salts [ 188 – 191 ] or other forms of creatine like effervescent creatine [ 128 ], creatine ethyl ester [ 43 , 192 , 193 ], buffered creatine [ 41 ], creatine nitrate [ 194 , 195 ], creatine dipeptides, or the micro amounts of creatine contained in creatine serum [ 196 ] and beverages (e.g., 25–50 mg) increases creatine storage in muscle to a greater degree than creatine monohydrate [ 187 ]. In fact, most studies show that ingestion of these other forms have less physiological impact than creatine monohydrate on intramuscular creatine stores and/or performance and that any performance differences were more related to other nutrients that creatine is bound to or co-ingested with in supplement formulations. This makes sense given that these other forms contain less creatine per gram than creatine monohydrate and that 99% of ingested creatine monohydrate is absorbed into the blood, then taken up into muscle, or excreted in urine [ 187 ].
Creatine monohydrate crystallizes from water as monoclinic prisms that hold one molecule of water of crystallization per molecule of creatine [ 187 ]. Subsequent drying of creatine monohydrate at about 100°C removes the water of crystallization yielding anhydrous creatine (100% creatine) [ 187 ]. Creatine is considered a weak base (pKb 11.02 at 25°C) that can only form salts with strong acids (i.e., pKa < 3.98). Creatine can also serve as a complexing agent with other compounds via ionic binding. Creatine monohydrate powder contains the highest percentage of creatine (87.9%) other than creatine anhydrous [ 187 ]. Creatine monohydrate manufactured in Germany involves adding acetic acid to sodium sarconsinate, heating, adding cyanamide, cooling to promote crystallization, separation and filtration, and drying has been reported to produce 99.9% pure creatine monohydrate with no contaminants. Meanwhile, other sources of creatine monohydrate that have different starting materials (e.g., sarcosinates and O-alkylisourea, sarcosinates and S-alkylisothiourea) and methods of creatine synthesis, particularly from sources produced in China, have been found to contain up to 5.4% dicyandiamide, 0.09% dihydrotriazine, 1.3% creatinine, dimethyl sulphate, thiourea, and/or higher concentrations of heavy metals like mercury and lead due to use of different chemical precursors, poorly controlled synthesis processes, and/or inadequate filtration methods that more readily produce these contaminants [ 197 ]. While the effects of ingesting these compounds on health are unknown, contamination with dihydrotriazine has been suggested to be of greatest concern since it is structurally related to carcinogenic compounds [ 197 ]. For this reason, German sourced creatine monohydrate has been primarily used in research to establish safety and efficacy and is therefore the recommended source of creatine monohydrate to use in dietary supplements [ 2 , 187 ].
Creatine monohydrate powder is very stable showing no signs of degradation into creatinine over years, even at elevated storage temperatures [ 187 ]. However, creatine is not stable in solution due to intramolecular cyclization that converts creatine to creatinine especially at higher temperatures and lower pH [ 187 , 198 – 200 ]. The degradation of creatine can be reduced or halted by lowering the pH under 2.5 or increasing the pH above 12.1 [ 187 ]. This is the reason that less than 1% of creatine monohydrate is degraded to creatinine during the digestive process and creatine is taken up by tissue or excreted in urine after ingestion [ 60 , 185 – 187 ]. Moreover, since creatine is an ampholytic amino acid, it is not very soluble in water (e.g., creatine monohydrate dissolves at 14 g/L at 20°C with a neutral pH of 7) [ 187 ]. Mixing creatine in higher temperature solution increase solubility, which is the reason why initial studies administered creatine in hot tea [ 35 , 60 , 103 , 104 , 123 , 182 ] but the solubility has no influence on tissue uptake [ 187 ]. The lack of solubility and stability of creatine in solution is the reason that creatine is primarily marketed in powder form and efforts to develop stable beverages containing physiologically effective doses of creatine (e.g., 3–5 g per serving) have been unsuccessful.
In summary, while some forms of creatine may be more soluble than creatine monohydrate when mixed in fluid, evidence-based research clearly shows creatine monohydrate to be the optimal choice.
Conclusions
Based on our evidence-based scientific evaluation of the literature, we conclude that:
- Creatine supplementation does not always lead to water retention.
- Creatine is not an anabolic steroid.
- Creatine supplementation, when ingested at recommended dosages, does not result in kidney damage and/or renal dysfunction in healthy individuals.
- The majority of available evidence does not support a link between creatine supplementation and hair loss / baldness.
- Creatine supplementation does not cause dehydration or muscle cramping.
- Creatine supplementation appears to be generally safe and potentially beneficial for children and adolescents.
- Creatine supplementation does not increase fat mass.
- Smaller, daily dosages of creatine supplementation (3-5 g or 0.1 g/kg of body mass) are effective. Therefore, a creatine ‘loading’ phase is not required.
- Creatine supplementation and resistance training produces the vast majority of musculoskeletal and performance benefits in older adults. Creatine supplementation alone can provide some muscle and performance benefits for older adults.
- Creatine supplementation can be beneficial for a variety of athletic and sporting activities.
- Creatine supplementation provides a variety of benefits for females across their lifespan.
- Other forms of creatine are not superior to creatine monohydrate.
Acknowledgments
Abbreviations, authors’ contributions.
Conceptualization: DGC; Writing-original draft preparation: All authors. The authors declare that the content of this paper has not been published or submitted for publication elsewhere. The author(s) read and approved the final manuscript.
Availability of data and materials
Ethics approval and consent to participate, consent for publication.
Not applicable
Competing interests
JA is Chief Executive Officer of the ISSN, an academic non-profit that receives support and/or sponsorship from companies that manufacture and/or sell creatine or creatine-containing products.
DGC has received research grants and performed industry sponsored research involving creatine supplementation, received creatine donation for scientific studies and travel support for presentations involving creatine supplementation at scientific conferences. In addition, DGC serves on the Scientific Advisory Board for Alzchem (a company which manufactures creatine) and the editorial review board for the Journal of the International Society of Sports Nutrition and is a sports science advisor to the ISSN. Furthermore, DGC has previously served as the Chief Scientific Officer for a company that sells creatine products.
SCF has served as a scientific advisor for a company that sells creatine products.
BG has received research grants, creatine donation for scientific studies, travel support for participation in scientific conferences (includes the ISSN) and honorarium for speaking at lectures from AlzChem (a company which manufactures creatine). In addition, BG serves on the Scientific Advisory Board for Alzchem (a company that manufactures creatine).
ARJ has consulted with and received external funding from companies that sell certain dietary ingredients and also writes for online and other media outlets on topics related to exercise and nutrition
RBK is co-founder and member of the board of directors for the ISSN. In addition, RBK has conducted industry sponsored research on creatine, received financial support for presenting on creatine at industry sponsored scientific conferences (includes the ISSN), and served as an expert witness on cases related to creatine. Additionally, he serves as Chair of the Scientific Advisory Board for Alzchem that manufactures creatine monohydrate.
ESR serves on the Scientific Advisory Board for Alzchem (a company which manufactures creatine). In addition, ESR received financial compensation to deliver the President’s Lecture on creatine supplementation at the 2019 ISSN annual conference.
AESR has received research funding from industry sponsors related to sports nutrition products and ingredients. In addition, AESR serves on the Scientific Advisory Board for Alzchem (a company that manufactures creatine).
TAV has received funding to study creatine and is an advisor for supplement companies who sell creatine. In addition, TAV is the current president of the ISSN.
DSW serves as a scientific advisor to the ISSN and on the editorial review board for the Journal of the International Society of Sports Nutrition. In addition, DSW is Past President of the ISSN and has received financial compensation from the ISSN to speak about creatine supplementation.
TNZ has conducted industry sponsored research involving creatine supplementation and has received research funding from industry sponsors related to sports nutrition products and ingredients. In addition, TNZ serves on the editorial review board for the Journal of the International Society of Sports Nutrition and is Past President of the ISSN.
Publisher’s Note
Springer Nature remains neutral with regard to jurisdictional claims in published maps and institutional affiliations.
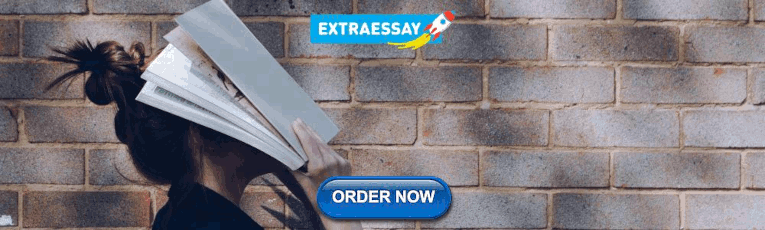
IMAGES
VIDEO
COMMENTS
The Case. A 42-year-old Caucasian man was referred because of proteinuria and decreased renal function. He had developed hypertension several years earlier and was being treated with hydrochlorothiazide and telmisartan. The heart rate was 64/min, while the blood pressure was 146/90 mm Hg. The personal and family histories were negative.
The nephron is the functional unit of the kidney. The glomerulus is a key regulator of filtration rate and filtration selectivity. Progression of chronic kidney disease is commonly monitored using glomerular filtration rate and albuminuria. Numerous laboratory methods exist to evaluate glomerular filtration rate.
Presentation of Case. Dr. Eugene P. Rhee: A 74-year-old man was evaluated in the nephrology clinic of this hospital because of chronic kidney disease. The patient had been in his usual state of ...
The kidneys play a vital role in the excretion of waste products and toxins such as urea, creatinine and uric acid, regulation of extracellular fluid volume, serum osmolality and electrolyte concentrations, as well as the production of hormones like erythropoietin and 1,25 dihydroxy vitamin D and renin. The functional unit of the kidney is the nephron, which consists of the glomerulus ...
level of cystatin is a better test for kidney function (GFR) than serum creatinine levels. Since, there is ... Clinical Case Study 25.2 Answer. The patient is suffering from acute renal failure (ARF),
M.D. Resources. Self eval. Case #1. The patient is a 41 year-old male who has a longstanding history of hypertension and diabetes and presents with a complaint of pruritis, lethargy, lower extremity edema, nausea and emesis. He denies any other medical illnesses. On physical exam the patient is a well-developed, well-nourished male in moderate ...
Abstract. The prevalence of chronic kidney disease and its risk factors is increasing worldwide, and the rapid rise in global need for end-stage kidney disease care is a major challenge for health systems, particularly in low- and middle-income countries. Countries are responding to the challenge of end-stage kidney disease in different ways ...
In this case, the home setting test of capillary blood is considered the baseline and is included in the ... Our study found that selected patients can be trained to use a POCT safely to self-test kidney function at home. Study participants had a similar test success rate at home (42/60, 70%, tests successful) to nurses in clinic (44/60. 73% ...
Kidney function tests measure how efficiently your kidneys are working. Most of these tests check how well your kidneys clear waste from your system. A kidney test may involve a blood test, 24-hour urine sample or both. You usually have your test results the same day or within a few days. Contents Overview Test Details Results and Follow-Up.
Several diseases, such as acute nephrotic syndrome, acute glomerulonephritis, and others, can singly or in combination precipitate renal diseases. Various clinical laboratory tests are useful in the investigation and evaluation of kidney function. Different treatment options available for the management and prevention of renal disease ...
An exploration of chronic kidney disease (CKD) focusing on a patient's experience on. admission to a nephrology ward. Causes of the disease include hypertension, diabetes. and acute kidney injury, and progression could be slowed by medication to reduce. blood pressure and glucose levels, along with dietary and lifestyle changes.
The case study of ARF is discussed to develop a better understanding by studying the current research project. The discussion will be more focused on the pathophysiology and the new ways of treatment used in current practice. ... Kidney function improved progressively, with creatinine level decreasing to 1.5 mg/dl during the course of 2 weeks. ...
Abstract. Exercise-induced acute kidney injury (EIAKI) frequently develops in patients with renal hypouricemia (RHUC). However, several cases of RHUC with acute kidney injury (AKI) but without intense exercise have been reported. We encountered a 15-year-old male with RHUC who experienced AKI. He reported no episodes of intense exercise and displayed no other representative risk factors of ...
A case study explores patterns of kidney function decline using unsupervised learning methods first and then associating patterns with clinical outcomes using supervised learning methods. Predicting short-term risk of hospitalization and death prior to renal dialysis initiation may help target high-risk patients for more aggressive management.
Albumin is an important protein in the blood. If the kidneys filter too much albumin out in the urine, it may be a sign that they are not doing their job correctly. A urine albumin result of 30 ...
Assessment and diagnosis of a patient with ARF include evaluation for changes in the urine, diagnostic tests that evaluate the kidney contour, and a variety of normal laboratory values. Urine. Volume: Usually less than 100 mL/24 hr (anuric phase) or 400 mL/24 hr (oliguric phase), which occurs within 24-48 hr after renal insult.
This case presents an elderly man with type 2 diabetes and impaired renal function. He has recently been experiencing hypoglycemia with his current treatment regimen. The importance of re ...
The left renal pelvis is slightly prominent compared to the right. Normal emptying of radiotracer from the upper urinary tract and ureters. Normal frusemide clearance half times of 7 minutes on the left, and 4 minutes on the right (normal < 10 minutes). The differential renal function at 2-3 minutes is symmetrical at 50% on the left and 50% on ...
Chronic kidney disease (CKD) is a non-communicable disease that includes a range of different physiological disorders that are associated with abnormal renal function and progressive decline in glomerular filtration rate (GFR). This study aimed to investigate the associations of several behavioral and health-related factors with CKD in Iranian ...
Background Chronic kidney disease (CKD) is a non-communicable disease that includes a range of different physiological disorders that are associated with abnormal renal function and progressive decline in glomerular filtration rate (GFR). This study aimed to investigate the associations of several behavioral and health-related factors with CKD in Iranian patients. Methods A hospital-based case ...
Urine tests. One of the earliest signs of kidney disease is when protein leaks into your urine (proteinuria). Urine testing can check for this. There are two types of urine tests that can check your protein levels. Dipstick urine test. A dipstick is a chemically treated paper placed in your urine sample. It changes color if your levels are ...
In this case, the home setting test of capillary blood is considered the baseline and is included in the ... Our study found that selected patients can be trained to use a POCT safely to self-test kidney function at home. Study participants had a similar test success rate at home (42/60, 70%, tests successful) to nurses in clinic (44/60. 73% ...
Abstract. Different biochemical markers exist in both blood and urine for assessing renal function. Most of these biomarkers have advantages and limitations associated with their use, which is important to consider when ordering and utilising them in the clinical setting. The ideal marker should be able to detect acute kidney injury (AKI) at ...
In a review of creatine supplementation studies, Persky and Rawson found no increase in serum creatinine in 12 studies, 8 studies showed an increase that remained within the normal range, and only 2 studies showed an increase above normal limits (although not different from the control group in one study). In 1998, a case study of a young male ...