- Search Menu
- Advance Articles
- Author Guidelines
- Open Access
- Submission Site
- Self-Archiving Policy
- About Journal of Geophysics and Engineering
- About Sinopec Geophysical Research Institute Co., Ltd
- Editorial Board
- Advertising and Corporate Services
- Journals on Oxford Academic
- Books on Oxford Academic

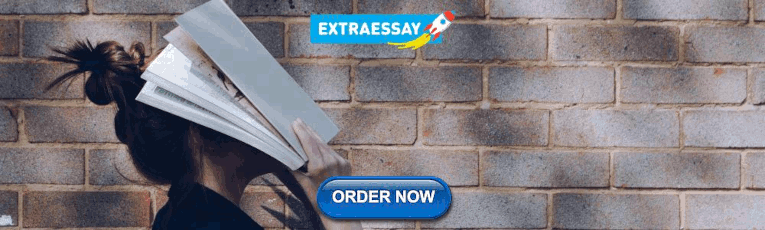
Article Contents
1 introduction, 2 area of investigation, 3 geophysical exploration, 4 geophysical measurements, 5 effect of clay on resistivity/conductivity measurements, 6 combinative geophysical interpretation, 7 real geological setting—comparison with geophysical results, 8 discussion—effect of clay on the foundation, 9 conclusions, acknowledgments.
- < Previous
Use of engineering geophysics to investigate a site for a building foundation
- Article contents
- Figures & tables
- Supplementary Data
P M Soupios, P Georgakopoulos, N Papadopoulos, V Saltas, A Andreadakis, F Vallianatos, A Sarris, J P Makris, Use of engineering geophysics to investigate a site for a building foundation, Journal of Geophysics and Engineering , Volume 4, Issue 1, March 2007, Pages 94–103, https://doi.org/10.1088/1742-2132/4/1/011
- Permissions Icon Permissions
The combination of geophysical data and geotechnical measurements may greatly improve the quality of buildings under construction in civil engineering. A case study is presented here at a vacant building site. Initially, boreholes indicated a complex geology. A dipole–dipole configuration was selected for electrical resistivity tomography (ERT) implementation and the data were processed and interpreted by applying 2D and 3D inversions. An electromagnetic survey was also carried out at a different time periods and successfully used to verify the results of the resistivity measurements. It is demonstrated that engineering geophysics is able to provide solutions for determining subsurface properties and that different prospection techniques are necessary for developing a reasonable model of the subsurface structure.
In the last decade, the involvement of geophysics in civil and environmental engineering has become a promising approach. Geophysical methods are implemented in a wide range of applications ranging from building ground investigations to the inspection of dams and dikes (Klimis et al 1999 , Luna and Jadi 2000 , Othman 2005 , Savvaidis et al 1999 , Soupios et al 2005 , 2006 , Venkateswara et al 2004 ), aiming towards the exploration of geological structures and the determination of the physical parameters of the rock formations. In engineering geophysics, the question of the quality of building foundations is frequently addressed in the very late stages, when earthquake damage is either observed or expected (Delgado et al 2000a , 2000b , Seht Malte Ibs-von and Wohlenberg 1999, Parolai et al 2001 , 2002 , Delgado et al 2002 ). In the case of building construction, geophysics can be applied for exploration purposes to provide useful information regarding the early detection of potentially dangerous subsurface conditions. The sources of hazards in civil engineering disciplines result essentially from undetected near-surface structures, such as cavities and/or inhomogeneities in the foundation geomaterials. Information related to the local soil conditions is vital for risk assessment and mitigation.
The procedure for obtaining subsurface information is divided into two broad categories: indirect and direct methods. Indirect methods include aerial photography, topographic map interpretation and the study of existing geological reports, maps, and soil surveys. Direct methods are comprised of the following modules: (a) geologic field reconnaissance, including the examination of in situ materials, man-made structures, groundwater level and exploration of shafts, (b) application of modern geophysical techniques for mapping subsurface structures, (c) borings, test pits, trenches and shafts from which representative disturbed and/or undisturbed samples of the in situ materials may be obtained and analysed and (d) simple geotechnical field tests, such as the standard penetration test (SPT), which can be correlated with other engineering parameters.
The above methodologies were applied in our case study within the urban context of the city of Hania, on the island of Crete, Greece. Two different geophysical methods were applied, namely, electrical resistivity tomography (ERT) (Ward 1990 ) and electromagnetic terrain conductivity (ETC), using the Syscal Jr Switch 48 channels by IRIS and the EM31-MK2 instruments by Geonics Limited, respectively. Resistivity profiling and electromagnetic mapping have been carried out in grids of 8 × 20 and 20 × 20 m, respectively. The resulting measurements were combined with the available geological and geotechnical reports of the study area and were used to assess the structure of the near-surface geology. After the acquisition of the geophysical data, interpretation of the resistivity results was carried out using 2D and 3D algorithms (Loke and Barker 1996 , Loke 1997 ) and confirmed by comparison to the corresponding electromagnetic map.
Excavations in the framework of the construction works that followed the geophysical survey confirmed that geophysical modelling could successfully approach the actual geological and geotechnical soil conditions. Moreover, the particular data obtained through indirect geophysical techniques contributed to the decision making process for choosing the appropriate footing of the structure based on the subsurface inhomogeneity of the site.
2.1 Location
The study area is located almost 200 m away from the premises of the Technological Educational Institute (TEI) of Crete in the Halepa area, an eastern suburb of Hania city, in western Crete. The area is bounded to the north by the streambed and, in its vicinity, a man-made shaft (possibly a hand-dug well) was found (figure 1 ). Similar features exist in the surrounding area, suggesting a shallow water table which usually causes severe structural problems. The surveyed area is near a stream denoted by the dashed line in figure 1 .

Satellite image map from Quickbird, showing the area where geophysical measurements were carried out in 2004. The dashed line denotes the streambed, the rectangle south of the stream shows the location of the surveyed site and the circle depicts a shaft.
2.2 Geological features
The city of Hania is laid over a complex geological structure, due to dense faulting of the whole area. Old faulting with E–W orientation has resulted in the creation of a small dipping basin, where nowadays the centre of the city is located. This basin is filled with Quaternary deposits covering most of the centre of the city and the southern part of it. Alluvial deposits, sand and anthropogenic deposits are evident in the northern part. As we move towards the south, marls appear at a few metres depth, covering most of the area in the south and east parts of the city. In the south-east part, massive Triassic limestone appears, which is considered the basement for the whole urban area. The limestone is dipping towards the north and the west at a few hundred metres depth.
Specifically, in the area under investigation, the geological formations are formed by alluvial, Quaternary and neogenic clay sediments, sands and roundstones. The sediments cover the biggest part of the stream banks but an olistolith (cohesive marly limestone conglomerates) has also been found in the south bank and in the study site. The stream in this area enters into an autochthonous alpine marly and dolomitic limestone overlain by alluvium, with an average thickness of 10 m, reaching 20 m in a few places. The drainage pattern of the area is closely related to the structural lines, with a general directional trend almost from east to west. Topographically, the area is in a mature stage as far as the fluvial erosion cycle is concerned.
From a hydrogeological point of view, the free underground water level is located 5 to 10 m below the surface (due to the adjacent stream), varying seasonally. The pore water pressure is hydrostatic from this level.
2.3 Preliminary explorations
The depth, thickness and the extent of all major soil and rock strata that will be affected by future construction must be determined in reasonable detail. The primary objective of these explorations is to obtain sufficient subsurface data to permit the reliable determination of the types, locations and principal dimensions of all major structures. Disturbed and undisturbed samples of the foundation and buried materials must be obtained for laboratory testing to provide a basic knowledge of their engineering properties. Thus, the research procedure requires a few reconnaissance drill holes that will verify the initial assumptions as to the type of geomaterial present, and the depth and thickness of the deposit. Then it will be possible to design a suitable framework including the prospecting depth.
The geotechnical properties of the ground were investigated by continuous core sampling of four boreholes drilled to depths of up to 16 m. The samples were obtained throughout the entire length of the hole and SPTs (standard penetration tests) were made at every change of geomaterial. The generalized soil profiles and geotechnical properties at these four boreholes, called B3, B3E, B1 and B2 respectively, are shown in figure 2 .

Geological/geotechnical profile of the study site based on borehole data.
The geological profile indicates stiff to hard silty sand, sand–silt mixtures (SM) up to a depth of 2 m, inorganic clays of low to medium plasticity, silty clays, gravelly and sandy clays, silty gravels, gravel-sand–silt mixtures (CL-GM) at depths from 2 to 4 m, inorganic silts and very fine gravels and sands (CL-ML) at depths from 4 to 8 m and finally inorganic silts and very fine gravels and sands, rock fragments and olistolith (ML) at depths from 8 to 16 m. The samples have been characterized according to the Unified Soil Classification System (USCS system).
The observed horizontal and vertical discontinuities of these strata are remarkable, which may be attributed to reworking by earlier streams. In order to resolve the ambiguities raised by irregularities in geological/geotechnical profiles and to achieve well-defined upper subsurface geological conditions, two geophysical methods were suggested as necessary additional explorations.
It should be mentioned that every geotechnical in situ survey gives only discontinuous one-dimensional information for the subsurface conditions. In contrast, the application of engineering geophysics can provide either 2D or 3D subsurface images of the study area.
Both ERT and ETC have repeatedly fulfilled the expectations for obtaining rapid and cost-effective subsurface information and are thus indispensable supplements to borings in exploratory surveys for civil engineering purposes (ASCE 1996 , Sharma 1997 ). These geophysical engineering tools provide valid information for bedrock profiles and yield a definite determination of the general subsurface structure including the depth of aquifers. Ideally, the resulting geophysical model should be combined with the results from borings and/or other direct methods of geotechnical and geological exploration in order to refine the interpretation of the geophysical measurements, which in some cases have limited success under specific soil conditions.
3.1 Electrical resistivity tomography (ERT)
Electrical resistivity tomography data are collected along a line as a combined sounding–profiling survey, using a multi-electrode resistivity measurement system. This data set can be inverted for the true subsurface resistivity by using 2D or 3D inversion algorithms and the resulting estimated models can be interpreted accordingly (Papadopoulos et al 2006 ). Electrical resistivity tomography is a method adapted to the recognition and study of 2D structures perpendicular to the profile and to the identification of the various geological layers, including the surface cover, but is more expensive and time consuming than the electromagnetic survey.
3.2 Electromagnetic terrain conductivity (ETC)
ETC surveys are especially adapted to map lateral variations of resistivity or conductivity distribution. Possible geological targets are steep dipping structures, such as faults and fracture zones. In particular, EM31 instrumentation features a large effective prospection depth (∼4–6 m), depending on the geoelectrical properties of the subsoil. The EM31 induces currents to the ground by emitting a 9.8 kHz electromagnetic field. The basic operation principles of the EM31 are outlined by McNeill ( 1980 , 1990 ) and Nobes ( 1999 ), which discuss the role of the orientation of the EM31 relative to certain targets. Analysis of the measured quadrature mode permits the estimation of the soil conductivity, whereas the analysis of the measured real mode estimates the magnetic susceptibility of the soil. It is emphasized that the vertical orientation of the coils is characterized by a greater penetration depth than the horizontal orientation.
4.1 Data acquisition and processing
The geoelectrical data were collected using an IRIS-Syscal Jr Switch 48 instrument with an accuracy of 0.1 mV. The system features 48 electrodes, enabling fully automated measurements of the shallow subsurface apparent resistivity using the dipole–dipole configuration. This technique has the advantage of very good horizontal resolution, but its main disadvantage is the relatively low signal strength (Sasaki 1992 ). Five geoelectrical profiles were carried out forming a grid with 2 m profile spacing (figure 3 , lines 1–5). Two additional profiles (lines 6 and 7), perpendicular to the previous ones, were conducted in order to verify the results. The dipole–dipole spacing ( a ) was 1 m, providing the possibility of detecting small bodies and/or structures up to 3 m depth, which may be considered satisfactory for the detection of near-surface geotechnical anomalies in the study area.

Topographic scheme of the study area. Circles (B#) depict the geotechnical boreholes, the dashed rectangle represents the area which was scanned using the ETC method and the other five arrows together with the two perpendicular ones indicate the geoelectrical tomography transects. Shaded polygons (F1–F4) indicate the places where buildings will be constructed.
A Geonics Limited EM31-MK2 instrument was used for the ETC survey. The prospection mode was set to carry out measurements in parallel profiles utilizing a 20 m × 20 m measuring grid. Readings were taken at 1 m intervals along parallel profiles also with 1 m spacings, achieving high-resolution mapping. The measurement layout is depicted in figure 3 (see the dashed line grid). Due to the existence of buildings in the investigated area, a limited number of choices were available concerning the location and orientation of the measuring grid. Furthermore, de-spiking algorithms were applied to filter out extreme values that aggravated the identification of interesting anomalies. Line equalization techniques in both dimensions ( X , Y ) were implemented for the rectification of the data to the mean-level base line. Application of filtering was crucial in cases where data suffered from instrumental and/or geological noise.
The resistivity cross section data were processed and inverted using the commercial packages RES2DINV (Loke 1997 ) and RES3DINV (Loke and Barker 1996 ). These programs use an implementation of the smoothness-constrained least-squares method (Sasaki 1989 ) based on the Gauss–Newton optimization technique. The 2D and 3D electrical responses are calculated either with the finite-difference or the finite-element method.
Each resistivity cross section was processed independently. Identical inversion parameters were used to process all the cross sections in order to reduce the misfit as much as possible. The good quality of the collected data resulted in quite low RMS errors for the inverted resistivity sections (below 7%).
The independent five parallel 2D lines were then combined into a single data set and the commercial software RES3DINV (Loke and Barker 1996 ) was used to produce the horizontal depth slices through the earth. The 3D model consisted of 643 model parameters. The inversion process converged to an acceptable solution after six iterations, while the resulting model had a percentage RMS error equal to 5.95%.
The presentation of the modelling results was made by means of the Surfer™ software that produced a plan-view image of the site's conductivity based on the analysis of the collected data.
4.2 Data interpretation
4.2.1 2d-inversion geoelectrical modelling.
The geoelectrical measurements were carried out along five parallel profiles spaced 2 m apart (figure 4 ). It must be stressed here (and will be commented on in a following section) that the geoelectrical survey was conducted during a separate field season (the summer of 2004). These profiles (so called lines 1 to 5) can be considered to be located at points 9.58, 7.58, 5.58, 3.58 and 1.58 m along the y -axis, while the measurements were conducted along the x -axis, where the xy -coordinate system is that of the electromagnetic survey.

Inversion model results inferred from electrical resistivity tomography profiles. RMS error fluctuates between 1.34% and 7.3%. Electrode spacing was 1 m. Black dashed lines, across the five parallel cross sections, indicate the position and direction of the two additional geoelectrical profiles conducted and the two resulting cross sections, as shown in the lower part of the figure.
The resistivity cross sections resulting from the inversion analysis of the geoelectrical data exhibit significant variations of resistivity values especially at shallow depths, as figure 4 depicts. We draw attention to an important underground anomaly characterized by low resistivity values (between 20 to 40 Ω m), which is recognizable in all sections at a distance ranging from 7.5 to 9.5 m on the x -axis and, moreover, at a distance ranging from 4 to 7 m on the y -axis. Based on the shape of this feature and the existence of similar structures in the broader area, this body could be reasonably identified as a possible hand dug well which has since filled with clay. Usually, clays exhibit low resistivities that range from 3 to 50 Ω m and sandy clays range from 10 to 100 Ω m.
Two additional geoelectrical profiles were carried out in perpendicular directions (which are indicated with dashed lines in figure 4 and shown in detail in the lower part of the figure) to verify the results from the first cross sections. The final inversion model for the latter two profiles reveals the same anomaly at 4 m and 13.5 m, respectively. Furthermore, between 20 m and 23 m on the y -axis, a linear high resistivity anomaly is detected which is interpreted as a stone wall foundation, shown as a dashed ellipse in figure 4 .
4.2.2 3D-inversion geoelectrical modelling
The dipole–dipole electrical resistivity pseudosection data sets collected along five parallel lines are inverted by using the 3D commercial inversion software RES3DINV (Loke and Barker 1996 ). The estimated model is represented as six different depth slices (figure 5 ). Their depths are 0.00–0.35 m, 0.35–0.75 m, 0.75–1.22 m, 1.22–1.75 m, 1.75–2.36 m and 2.36– 3.06 m, respectively. The two upper (shallow) slices (see figure 5 ) indicate strong variations in resistivity values (20 to 800 Ω m) attributable to the shallow inhomogeneities of the soil and rock material present, as depicted in figure 2 . The remaining deeper slices reveal a low resistivity anomaly with coordinates of 9 m on the x -axis and 7 m on the y -axis, which could be safely correlated with the man-made shaft as mentioned above.

3D inversion model of the electrical resistivity tomography profiles. Iteration: 6 and RMS error: 5.95%.
4.2.3 Electromagnetic survey results
The electromagnetic survey was carried out in autumn 2004. Both geophysical measurements should be acquired in the same time period in order to have comparable results. Unfortunately, the EM equipment was not available in the summer period and additionally, during the autumn period (when the EM measurements were carried out) limited access to the site was available since construction work at the site had been initiated.
The composite map image (figure 6 ) is a 2D-colour scale map of the shallow subsurface apparent conductivity in mS m -1 from the EM31 quadrature data. It should be reported that very significant interference was experienced in the beginning (first 5 m along the x -axis) of all the electromagnetic profiles due to the various buildings and spoil metal fragments present at this side of the study site. The circular shaped low conductivity anomaly, located at x = 9 m, y = 6.5 m, is closely correlated with the low resistivity anomaly which had been determined by the electrical resistivity tomographies after their 2D and 3D interpretation.

2D contour image of the soil conductivity resulting from the synthesis of the parallel EM transects. Measurements of conductivity in mS m -1 .
The dimensions of the particular anomaly is 4 × 3 square metres, consisting of low conductivity values reaching a minimum of 24.3 mS m -1 , surrounded by a background conductivity of 25.0–25.5 mS m -1 . Although the particular anomalous conductivity measurements are close to the threshold accuracy (±0.5 mS m -1 ) of the instrument at 20 mS m -1 level, the number of measurements that define the particular anomaly (more than 9) increase the confidence level of delineating the particular feature.
The apparent contradiction (low conductivity–low resistivity) for the same place is fully discussed and clarified in a following section below. Furthermore, another low conductivity zone is identified in the lower right part of the composite map image (figure 6 ), probably associated with the foundation of a stone wall, the surficial appearance of which is located at the bottom-right margin of the EM grid.
The apparent ambiguity, called low resistivity–low conductivity (LRLC), produced by the implementation of the two different geophysical methodologies and related to the above-mentioned detected geophysical anomaly, could be resolved in the context of the time difference between the conducted surveys. As mentioned in the sections above, the ERT measurements were carried out in summer of 2004, whereas EM data were collected in autumn of the same year. This inconformity drove the experimental work to examine the temporal stability of the results and their relative independence of the environmental conditions, by taking into account that the near-surface geological structure was studied.
The samples were measured at both high (8 kHz, similar to EM31 emitted electromagnetic field) and dc-like (1 Hz) frequencies, in order to testify that resistivity variations of the measured samples for various water contents exhibit similar behaviour at different frequencies.
Marly limestone samples of cylindrical shape (40 mm diameter and 8 mm thickness) remained in deionized water for a few days, resulting in a water saturation of approximately 2% ww. Clay samples were measured in powder form at low water contents, in order to correlate their resistivity values with those of marly limestone samples. All the samples were dried at 105 °C for sufficient time and the resistivity measurements were repeated again.
In order to associate the measured resistivity values with the effect of clay on resistivity/conductivity and the influence of water, it is useful to refer to the general climatic trends that exist in the study area. Specifically, the climatological conditions in Crete during summer are very dry with almost no precipitation, and intense irrigation lowers significantly the aquifer. Thus, during this period subsurface clay remains much more humid (because of the remaining pore water) from the surrounding gravels and marly limestones. Contextually, the detected anomaly is expected to exhibit much lower resistivity compared to the surrounding geology.
On the other hand, during autumn the rainy season begins, thus the soil becomes much more homogeneously conductive relative to its summer condition as the aquifer also rises. This is evident from the inspection of the conductivity values and their dispersion observed with EM measurements. However, the clay, due to its low plasticity, granularity and porosity, is only slowly moistened compared to the surrounding gravels and marly limestones, and thus appears less conductive.
The LRLC behaviour of the clay and the surrounding rocks is in agreement with the measured resistivities and the literature (Butler and Knight 1998 ). Dried clay is less resistive than marly limestone at zero water content (summer period), as is shown in figure 7 . As the water content in both samples increases (transition period from summer to autumn), the clay becomes more resistive than the marly limestone (see figure 7 ). This could be explained by the different amount of adsorbed water in each sample, as mentioned previously.

Log–linear plot of the water content (%) versus the specific resistance (Ω m) for the acquired samples (clay and marly limestones) for two different frequencies.
Summarizing, from the laboratory measurements above, it is clear that the difference between the two data sets (geoelectrical and electromagnetic) is due to the partial and overall seasonal change in resistivity of the clay material and its surrounding geology, being on average over twice as resistive in summer than in autumn. A small change in the amount of pore water (e.g. increased precipitation in autumn) can have a significant effect on the overall conductivity (McNeill 1990 ).
Geoelectrical resistivity 2D and 3D inversion modelling of the ERT data together with shallow subsurface apparent terrain conductivity contours from the EM survey were combined to enable a complementary and cross-validated interpretation. Both geophysical engineering methods indicate the presence of two major and consequently important geophysical anomalies (A1 and A2). The first one ‘low resistivity–high conductivity’ (A1) is denoted with a dotted ellipse in figure 8 and was determined from the ERT prospection. At almost the same position, the EM exploration defined a circular low conductivity–high resistivity anomaly.

Combined geophysical determinations from ERT and ETC data. The dashed ellipse (A1) represents the low and high geoelectrical resistivity anomalies from the ERT survey. The dashed rectangle defines the area of the low resistivity anomaly, A2 (stone wall).
The second identified anomaly (A2) is a high resistivity body that is depicted in the lower right corner of figure 8 (in the area of building F4). This anomaly seems to be continued as it is inferred from the results of the EM survey and the high resistivity anomalies depicted in the perpendicular inversion models (around location 22–26 m along lines 6 and 7, figure 4 ).
In October 2004, excavation commenced in the area, initiating the building construction. It was a great opportunity to verify the geophysical modelling results by comparing them with the actual geological conditions.
A 3 m thick discontinuity in marly limestone filled with clay was detected at the location of anomaly A1, by the end of the excavation works (figure 9 ). The geometrical regularity of this structure (e.g. vertical borders, invariant thickness) suggested that it was a hand dug well.

Excavation works revealed a disrupted part of the marly limestone, filled with red clay. The location of this geological–geotechnical inhomogeneity, coincides with that of the resistivity anomaly determined by the implementation of geophysical engineering methods.
It is emphasized that the structure was found at the exact location indicated by the geophysical measurements, and its geometrical characteristics were in agreement with the determined circular geophysical anomaly.
The dissimilarity which was observed in the geotechnical boreholes through the typical SPT approach required the complementary use of geophysical investigations in order to clarify the image of soil morphology and explain adequately the variance of the geotechnical rates. The main problem discovered from the geotechnical measurements and the later detailed geophysical study was the existence of clay in specific places.
The clay soils, even those which are not pre-stressed, i.e. when they are not under a long-term process of compression and stiffening (gradual reduction in pore fluid pressure and increase of the effective stress), are more susceptible to subsidence and they are also affected by environmental conditions (besides others), particularly temperature and moisture, which vary irregularly throughout the year. The results of the geotechnical boreholes showed that the specific type of clay, which was found in lenticular form, is very neogenic and soft (easy penetration: Nspt = 15–18), supporting our considerations about the nature of the clay in the site.
Likewise, it is recommended that continuous foundation footings should not be placed on soils comprised of clay alternating with stiffer soils. In such a case, the rate of the elastic ground coefficient, Ks , is not stable lengthwise and for this reason it is harder to support properly the entire foundation, with obvious consequences for the bearing structure. When this appearance of clay is restricted and has a very local nature, as it is here, then it is preferable to place the building elsewhere.
Engineering geophysics combined with geotechnical engineering focuses on the behaviour and performance of soils and rocks in the design and construction of civil, environmental and mining engineering structures.
Often, existing buildings are located over anomalous subsurface zones which are inappropriate for bearing the load of a structure. Moreover, building foundations may not have been built properly. Recent and present damage, such as terrain subsidence and cracks in houses, call for attention and for further investigation using some non-destructive geophysical methods to guide additional exploratory trenching and drilling.
Based on the above, geophysical surveys could contribute significantly in major construction projects in which subsurface structural problems are possible. The approach outlined in this paper allows for the rapid characterization of subsurface formations that can help to guide site investigations through the advancement of soil borings or test pits. Anomalous areas, as defined by the results of the geophysical surveys (such as electrical resistivity tomography and shallow electromagnetic terrain conductivity) in the early stage of construction work, can be used to prepare construction bid documents and estimate potential costs for in situ material handling and the possible reinforcement of the local complex geological structure for safe construction. All this information is vital to the successful completion of construction projects where development costs can be significant if site characterization does not adequately address the geological formation types and geotechnical parameters observed during the actual construction process.
The authors would like to thank the Skouloudis & Xaritakis Construction Company and D Diamantakis Technical Office for making available geotechnical data from the study area and for providing the license to measure in the area. We are also grateful to the students involved in the survey work: Giuseppe Mastrolorenzo, Evi Seferou and Maria Mixalaki.
Google Scholar
Google Preview
Email alerts
Citing articles via, affiliations.
- Online ISSN 1742-2140
- Print ISSN 1742-2132
- Copyright © 2024 Sinopec Geophysical Research Institute Co., Ltd
- About Oxford Academic
- Publish journals with us
- University press partners
- What we publish
- New features
- Open access
- Institutional account management
- Rights and permissions
- Get help with access
- Accessibility
- Advertising
- Media enquiries
- Oxford University Press
- Oxford Languages
- University of Oxford
Oxford University Press is a department of the University of Oxford. It furthers the University's objective of excellence in research, scholarship, and education by publishing worldwide
- Copyright © 2024 Oxford University Press
- Cookie settings
- Cookie policy
- Privacy policy
- Legal notice
This Feature Is Available To Subscribers Only
Sign In or Create an Account
This PDF is available to Subscribers Only
For full access to this pdf, sign in to an existing account, or purchase an annual subscription.
Analysis of damage evolution and study on mesoscopic damage constitutive model of granite under freeze–thaw cycling
- Original Paper
- Published: 16 May 2024
- Volume 83 , article number 236 , ( 2024 )
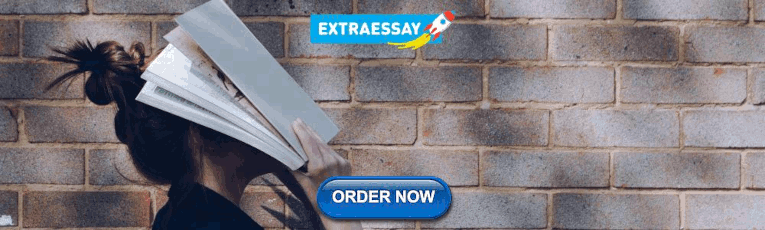
Cite this article
- Sibo Jia 1 ,
- Qingyang Yu 1 ,
- Huichao Yin 2 ,
- Zhenxue Dai 1 ,
- Shangxian Yin 3 ,
- Yimeng Kong 1 ,
- Hung Vo Thanh 4 , 5 , 6 &
- Mohamad Reza Soltanian 7
29 Accesses
Explore all metrics
The failure of rocks in seasonal frozen areas under freeze–thaw cycles (FTCs) is a frequently problem in engineering construction, posing a huge threat to the stability of the engineering. In order to explore the mechanism of rock damage degradation. It is necessary to analyze the damage evolution process of rocks and establish an accurate FTCs rock damage constitutive model. Taking the granite in the seasonal frozen zone of Northeast China as the research object, the macro and meso parameters and microstructure of the FTCs granite were analyzed through indoor tests. A new method is proposed to define damage variables considering mesoscopic parameters to establish a mesoscopic damage constitutive model for rocks, further revealing the damage mechanism and failure law of rocks under freeze–thaw load coupling. The research results indicate that in the early stage of FTCs, 20 cycles contribute significantly to the damage and deterioration of rocks, accounting for about 50% of the 80 cycles. After 20 cycles, the degradation trend of various macroscopic and mesoscopic parameters is relatively slow. The residual strain of the rock accumulates as the number of FTCs increases, the brittleness of the rock weakens, and the plasticity strengthens. Based on the established new method of considering mesoscopic parameter damage variables, the viewpoint of introducing correction coefficients based on statistical constitutive models has been validated. After uniaxial compression test data verification, the model has shown good performance. This model expands the damage model of rock freeze–thaw compression coupling effect, providing reference for research on FTCs related to rocks.
This is a preview of subscription content, log in via an institution to check access.
Access this article
Price includes VAT (Russian Federation)
Instant access to the full article PDF.
Rent this article via DeepDyve
Institutional subscriptions
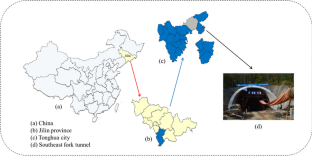
Similar content being viewed by others
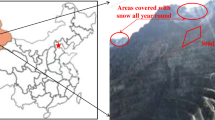
Research on macro and meso damage model of pre-flawed granites subjected to coupling action of freeze-thaw and loading
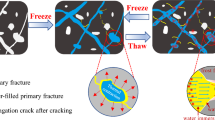
Investigation of the Constitutive Damage Model of Rock Under the Coupled Effect of Freeze–Thaw Cycles and Loading
Mechanical behavior and damage constitutive model of granite under coupling of temperature and dynamic loading, data availability.
This article introduces the available data generated during this study.
Amiri SAG, Grimstad G, Kadivar M et al (2016) Constitutive model for rate-independent behavior of saturated frozen soi-ls. Can Geotech J 53(10):1646–1657. https://doi.org/10.1139/cgj-2015-0467
Article Google Scholar
Ahmadi S, Ghasemzadeh H, Changizi F (2021a) Effects of a low-carbon emission additive on mechanical properties of finegrained soil under freeze-thaw cycles. J Clean Prod 304:127157. https://doi.org/10.1016/j.jclepro.2021.127157
Article CAS Google Scholar
Ahmadi S, Ghasemzadeh H, Changizi F (2021b) Effects of thermal cycles on microstructural and functional properties of nano treated clayey soil. Eng Geol 280:105929. https://doi.org/10.1016/j.enggeo.2020.105929
Chen RS, Kang ES, Wu LZ et al (2005) Discussion on the Distribution of Cold Regions in China [J]. Glacier Frozen so-Il 27(4):469–475 ( (in Chinese) )
Google Scholar
Chu Y, Zhang D, Song S, et al (2023) Experimental study on the evolution of pore structure of coal samples under freeze–thaw. Physics of Fluids 35(3). https://doi.org/10.1063/5.0145187
Freire-Lista D M, Fort R, Varas-Muriel M J (2015) Freeze–thaw fracturing in building granites. Cold Regions Science and Technology 113: 40–51. https://doi.org/10.1016/j.coldregions.2015.01.008
Ghobadi MH, Babazadeh R (2014) Experimental studies on the effects of cyclic freezing–thawing, salt crystallization, and thermal shock on the physical and mechanical characteristics of selected sandstones. Rock Mech Rock Eng 48(3):1001–1016. https://doi.org/10.1007/s00603-014-0609-6
Ghasemzadeh H, Ghoreishian Amiri SA (2013) A hydro-mechanical elastoplastic model for unsaturated soils under isotropic loading conditions. Comput Geotech 51:91–100. https://doi.org/10.1016/j.compgeo.2013.02.006
Ghasemzadeh H, Sojoudi MH, Ghoreishian Amiri SA et al (2017) Elastoplastic model for hydro-mechanical behavior of unsaturated soils. Soils Found 57(3):371–383. https://doi.org/10.1016/j.sandf.2017.05.005
Ghoreishian Amiri SA, Grimstad G, Kadivar M (2022) An elastic-viscoplastic model for saturated frozen soils. Eur J Environ Civ En 26(7):2537–2553. https://doi.org/10.1080/19648189.2016.1271361
Huang SB, Liu QS, Cheng AP, Liu YZ et al (2018) A statistical damage constitutive model under freeze-thaw and loading for rock and its engineering application. Cold Reg Sci Technol 145:142–150. https://doi.org/10.1016/j.coldregions.2017.10.015
Huang SB, Ye Y, Cui X et al (2020) Theoretical and experimental study of the frost heaving characteristics of the saturated sandstone under low temperature. Cold Reg Sci Technol 174:103036. https://doi.org/10.1016/j.coldregions.2020.103036
Inada Y, Yokota K (1984) Some studies of low temperature rock stength. Intl J RockMech Mining Sci Geomech Abstracts 21(3):145–153. https://doi.org/10.1016/0148-9062(84)91532-8
Jia H, Xiang W, Krautblatter M (2015) Quantifying Rock Fatigue and Decreasing Compressive and Tensile Strength after Repeated Freeze-Thaw Cycles. Permafrost Periglac Process 26:368–377. https://doi.org/10.1002/ppp.1857
Jia H, Ding S, Zi F et al (2020) Evolution in sandstone pore structures with freeze-thaw cycling and interpretation of damage mechanisms in saturated porous rocks. CATENA 195:104915. https://doi.org/10.1016/j.catena.2020.104915
Lemaitre J (1984) How to ues damage mechanics. Nucl Eng Des 80(3):233–245. https://doi.org/10.1016/0029-5493(84)90169-9
Liu QS, Xu XC (2000) Damage analysis of brittle rock under temperature[J]. J Rock Mech Eng 19(4):408–411 ( (in Chinese) )
Liu QS, Huang SB, Kang YS et al (2015) Research on rock mass freeze-thaw fatigue damage model and evaluation index[J]. J Rock Mech Eng 34(6):1116–1127 ( (in Chinese) )
CAS Google Scholar
Li SC, Xu J, Li KG (2007) Statistical constitutive model of rock damage based on the correction of initial damage coefficient[J]. J Sichuan Univ (Engineering Science Edition) 33(6):41–44 ( (in Chinese) )
Li B, Ren C, Wang Z et al (2020) Experimental study on damage and the permeability evolution process of methane-containing coal under different temperature conditions. J Petrol Sci Eng 184:106509. https://doi.org/10.1016/j.petrol.2019.106509
Li C, Jin J, Wu P et al (2022) Effect of Freeze-Thaw Cycles on Shear Strength of Tailings and Prediction by Grey Model. Minerals 12(9):1125. https://doi.org/10.3390/min12091125
Matsuoka H, Nakai T (1974) Stress-deformation and strength characteristics of soil three different principal stress. Pro-Ceedings of JSCE 2:59–70. https://doi.org/10.2208/JSCEJ1969.1974.232-59
Matsuoka H, Hoshikawa T, Ueno K (1990) A general failure criterion and stress-strain retain for granual materials to metals. Soil Found 30(2):119–127. https://doi.org/10.3208/sandf1972.30.2_119
Meng XZ, Zhang HM, Liu XY (2021) Rock damage constitutive model based on the modified logistic equation under freeze–thaw and load conditions. J Cold Regions Eng 35(4):04021016. https://doi.org/10.1061/(ASCE)CR.1943-5495.000026
Polak J (1991) Cyclic Plasticity and Low Cycle Fatigue Life of Metal. Elsevier, New York
Sondergeld CH, Rat CS (2005) Observations of velocity and resistivity changes during freeze-thaw cycles in Berea sand-stone. SEG Tech Program Expanded Abstracts 24(6):1509–1512. https://doi.org/10.1190/1.2147977
Satake M (1976) Stress-deformation and strength characteristics of soil three different principal stress(Discus-sion). Proc.of Japan Society of Civil Engineers 246:137–138.
Song CY (2018) Research and application of micro structural characteristics and deformation failure mechanism of weakly cemented sandstone[J]. J Rock Mech Eng 37(3):779 ( (in Chinese) )
Song YJ, Yang HM, Tan H et al (2021) Research on damage evolution characteristics of sandstone with different saturation under freezing and thawing environment[J]. J Rock Mech Eng 40(8):1513–1524 ( (in Chinese) )
Xu GM, Liu QS (2005) Analysis of rock freeze-thaw failure mechanism and experimental study on freeze-thaw mechanics[J]. J Rock Mech Eng 24(17):3076–3082 ( (in Chinese) )
Yang GS, Xi JM, Li HJ et al (2010) Experimental study on the characteristics of frozen Rock mechanics under three-dimensional stress[J]. J Rock Mech Eng 29(3):459–464 ( (in Chinese) )
Yu Q, Dai Z, Zhang Z et al (2018) Estimation of Sandstone Permeability with SEM Images Based on Fractal Theory. Transp Porous Media 126(3):0701–0712. https://doi.org/10.1007/s11242-018-1167-2
Yu Q, Xiong Z, Du C et al (2020) Identification of rock pore structures and permeabilities using electron microscopy experiments and deep learning interpretations. Fuel 268:117416. https://doi.org/10.1016/j.fuel.2020.117416
Yu Q, Lei P, Dai Z et al (2023) Damage Characteristics of Limestone under Freeze-Thaw Cycle for Tunnels in Seasonal Frozen Areas. Iranian J Sci Technol Trans Civil Eng 47(1):469–477. https://doi.org/10.1007/s40996-022-00979-7
Zhang QS, Yang GS, Ren JX (2003) A new approach to rock damage variables and constitutive equation[J]. J Rock Mech Eng 21(3):30–34 ( (in Chinese) )
Zhang HM, Lei LN, Yang GS (2014) Rock damage model based on Weibull statistical distribution[J]. J Hunan Univ Sci Technol (natural Science Edition) 29(3):29–32 ( (in Chinese) )
Zhang HM, Zhang MJ, Xie XM et al (2015) Experimental study on physical and mechanical properties of red sandstone under freeze-thaw cycle[J]. J Taiyuan Univ Technol 46(1):69–74 ( (in Chinese) )
Zhang HM, Wang YF (2022) Multi scale analysis of damage evolution of freeze-thaw red sandstone. Geotech Mech 43(8):2103–2114. https://doi.org/10.16285/j.rsm.2021.1726
Zhao T, Yang GS, Xi JM et al (2019) Experimental study on macro and meso freeze-thaw damage characteristics of Cretaceous sandstone[J]. J Xi’an Univ Sci Technol 39(2):241–248 ( (in Chinese) )
Zhou ST, Jiang N, Luo XD et al (2020) Uniaxial compression fractal damage constitutive model of rock subjected to freezing and thawing. Periodica Polytech Civil Eng 64(2):500–510. https://doi.org/10.3311/PPci.15313
Zhang Q, Li XC, Gao JX et al (2022) Macro-and Meso-Damage Evolution Characteristics of Coal Using Acoustic Emission and Keuence Testing Technique. Nat Resour Res 31:517–534. https://doi.org/10.1007/s11053-021-10006-7
Zhang Q, Li XC, Li B et al (2023) Continuous-Discontinuous Element Numerical Modeling of Damage and Fracture Characteristics of a Loaded Coal. Nat Resour Res 32:373–389. https://doi.org/10.1007/s11053-022-10147-3
Download references
Acknowledgements
This work is funded by the National Natural Science Foundation of China (NSFC: U2267217, 42141011, 42002254).
Author information
Authors and affiliations.
College of Construction Engineering, Jilin University, Changchun, 130026, China
Sibo Jia, Qingyang Yu, Zhenxue Dai & Yimeng Kong
Beijing and the School of Information Engineering, Institute of Disaster Prevention, China University of Mining and Technology, Langfang, 065201, China
Huichao Yin
North China Institute of Science & Technology, Langfang, 065201, China
Shangxian Yin
Laboratory for Computational Mechanics, Institute for Computational Science and Artificial Intelligence, Van Lang University, Ho Chi Minh City, Vietnam
Hung Vo Thanh
Faculty of Mechanical - Electrical and Computer Engineering, School of Technology, Van Lang University, Ho Chi Minh City, Vietnam
MEU Research Unit, Middle East University, Amman, Jordan
Departments of Geosciences and Environmental Engineering, University of Cincinnati, Cincinnati, OH, USA
Mohamad Reza Soltanian
You can also search for this author in PubMed Google Scholar
Corresponding author
Correspondence to Qingyang Yu .
Ethics declarations
Competing interest.
The authors declare no competing financial interest.
Rights and permissions
Reprints and permissions
About this article
Jia, S., Yu, Q., Yin, H. et al. Analysis of damage evolution and study on mesoscopic damage constitutive model of granite under freeze–thaw cycling. Bull Eng Geol Environ 83 , 236 (2024). https://doi.org/10.1007/s10064-024-03741-7
Download citation
Received : 19 July 2023
Accepted : 09 May 2024
Published : 16 May 2024
DOI : https://doi.org/10.1007/s10064-024-03741-7
Share this article
Anyone you share the following link with will be able to read this content:
Sorry, a shareable link is not currently available for this article.
Provided by the Springer Nature SharedIt content-sharing initiative
- Freeze–thaw cycle
- Microscopic
- Freeze–thaw damage variables
- SMP criteria
- Constitutive model
- Find a journal
- Publish with us
- Track your research
Academia.edu no longer supports Internet Explorer.
To browse Academia.edu and the wider internet faster and more securely, please take a few seconds to upgrade your browser .
Enter the email address you signed up with and we'll email you a reset link.
- We're Hiring!
- Help Center
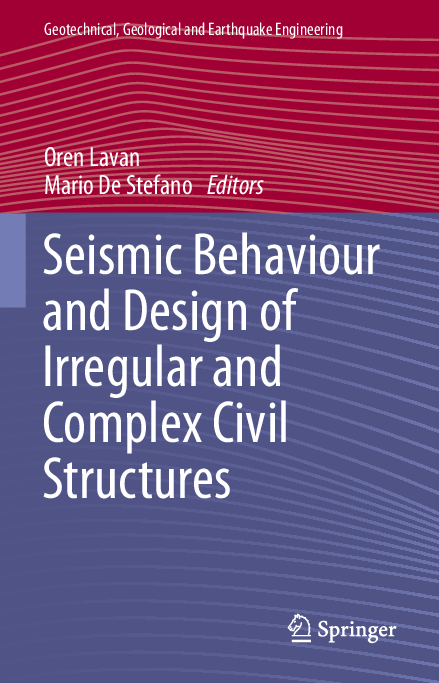
Geotechnical, Geological and Earthquake Engineering Seismic Behaviour and Design of Irregular and Complex Civil Structures

Related Papers
Ivan Hadi Santoso
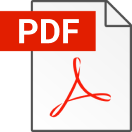
Geoenvironmental Disasters
Veronica Pazzi
Farzad Valipoor
Dharmapuram Reddy
Earthquake Engineering
Adem DOGANGUN
Gianni Niccolini
Abbas Moustafa
This special issue of Natural Science, entitled Earthquakes, tackles a wide range of research subjects related to earthquakes, including their nature, measures, causes of occurrence, characteristics, attenuation modeling, soil wave amplification, methods and tools of recording, analysis and prediction, their effect on the built environment and methods of design, analysis, assessment, control and repair of engineering structures against seismic loads resulting from earthquakes. This special issue of Natural Science considers also other related subjects on explosions and volcanic phenomena and hyperbaric-Oxygen treatment due to crush injury resulting from earthquakes. The special issue contains fourteen research papers written by researchers and experts from several countries.
Sassan Eshghi , Mehran Razzaghi
On 26 December 2003 an earthquake of magnitude Ms = 6.5 with a focal depth of about 8km occurred in southeastern Iran. The earthquake caused intense ground shaking throughout the affected area. Special structures such as on-grade steel oil tanks, elevated tanks, and industrial equipment were damaged during the earthquake. This paper presents the results of an investigation of the behavior of these special structures in Bam. Strong motion characteristics as recorded by accelerograms are discussed, as well as the failure modes of structures and components located within the affected area. An investigation into the response of an electrical transformer was carried out as a case study of a simple system.
hasan tosun
camillo Nuti
RELATED PAPERS
Extra limites
Artur Błażejewski
Music & Letters
Oliver Chandler
25th Annual European Real Estate Society Conference
Jurnal Ilmu Keperawatan dan Kebidanan
Ika Tristanti S.SiT.M.Kes
Radiation Protection Dosimetry
Sabine Mayer
Horticulturae
Montaser El-Hariry
Biosensors and Bioelectronics
Mara Mirasoli
Brazilian Journal of Medical and Biological Research
TANIA CAMILA DIAZ CASTRO
Preventive veterinary medicine
Revista Ingenieria E Investigacion
cesar collazos
Call Girls In Saket
Geta Sharma
IAHS Publications-Series of …
Nadhir Al-Ansari
Proceedings
Revista de Investigaciones Veterinarias del Perú
JHONY DE LA CRUZ-VARGAS
Meredith Hearshaw
Journal of agricultural and biological science
Abul Kashem
Journal of Biological Physics
Walter Sannita
Elizabeth Chavez
Eduardo Galhardo
Nila Michele Bastos Santos
OSH UNIVERSITY
OSH University
Cadernos de Sion
Luciano J O S É Dias
- We're Hiring!
- Help Center
- Find new research papers in:
- Health Sciences
- Earth Sciences
- Cognitive Science
- Mathematics
- Computer Science
- Academia ©2024
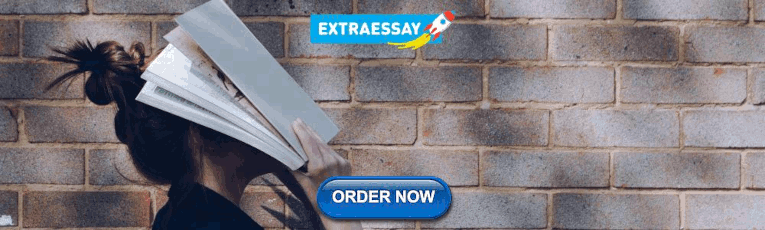
IMAGES
VIDEO
COMMENTS
Engineering Geology is an international interdisciplinary journal bridging the fields of the earth sciences and engineering, particularly geological and geotechnical engineering. The focus of the journal is on geological or engineering studies that are of interest to engineering geologists, …. View full aims & scope.
This Engineering geology research on rock mass classification to determine the support system's design and stand-up time at the Asahan 3 hydroelectric tunnel STA 7+085 - STA 7+717 has not been ...
Read the latest articles of Engineering Geology at ScienceDirect.com, Elsevier's leading platform of peer-reviewed scholarly literature ... Research Papers; Technical Notes; ... Article 106421 View PDF; Research Papers. select article An experimental investigation of shear strength behavior of a welded bimrock by meso-scale direct shear tests ...
Read the latest articles of Engineering Geology at ScienceDirect.com, Elsevier's leading platform of peer-reviewed scholarly literature ... Research Papers; Technical Note; Corrigendum; ... Full text access Editorial Board Article 107518 View PDF; Research Papers. select article Hydraulic properties of stressed granite fractures with heat ...
PDF | On Mar 18, 2020, Vassilis Marinos published Engineering Geology and Tunnels | Find, read and cite all the research you need on ResearchGate
1488 Book Review: Selected papers on engineering. geology and geotechnics 1. Our Italian colleagues have taken a valuable initiati ve in. selecting 20 of Professor Hutchinson's papers for ...
Covers a wide spectrum of applied interdisciplinary and multidisciplinary research, from geology to engineering; Presents a series of practical case studies and real-life experiences of researchers and practitioners from across the globe (5 continents / 16 countries) working in various geological, environmental and social settings
Engineering geological models: an introduction: IAEG commission 25. S. Parry, F. Baynes, +5 authors. D. Paul. Published in Bulletin of Engineering… 18 February 2014. Geology, Engineering. The generation and use of engineering geological models should be a fundamental activity for any geotechnical project. Such models are an essential tool for ...
Bulletin of Engineering Geology and the Environment - Aggregates from primary resources. Most of the construction aggregates (Table 1) still come from primary natural resources—natural sands and gravels, or crushed stone aggregates (Poulin et al. 1994; Smith and Collis 2001), although importance of secondary sources (industrial by-products or wastes, manufactured aggregates, construction and ...
Bulletin of Engineering Geology and the Environment is a comprehensive journal addressing issues related to geology and its implications in engineering and environmental contexts. Official journal of the International Association for Engineering Geology and the Environment. Reports on problems resulting from the interaction between geology and ...
Modelling non-isothermal volume change and solute transport behaviours of a semi-permeable clay soil under the combined influence of mechanical loading, chemical-osmosis, and thermo-osmosis. Zhihong Zhang, Shakil A. Masum, Gailei Tian, Hywel R. Thomas. Article 106271.
Geotechnical and Geological Engineering publishes papers in the areas of soil and rock engineering and also of geology as applied in the civil engineering, mining and petroleum industries.The emphasis is on the engineering aspects of soil and rock mechanics, geology and hydrogeology, although papers on theoretical and experimental advances in ground mechanics are also welcomed for inclusion.
Engineering geological. models can help to plan and developed the site investig ation activities as they constitut e. an idealization of the ground, quite useful during the office and field tasks ...
1 Introduction. In the last decade, the involvement of geophysics in civil and environmental engineering has become a promising approach. Geophysical methods are implemented in a wide range of applications ranging from building ground investigations to the inspection of dams and dikes (Klimis et al 1999, Luna and Jadi 2000, Othman 2005, Savvaidis et al 1999, Soupios et al 2005, 2006 ...
The Earth is a dynamic medium that changes and evolves through major events such as plate tectonics and earthquakes. Geologic time is a scale dividing the age of the Earth into five eras: Precambrian, Paleozoic, Mesozoic, Tertiary, and Quaternary. The Earth crust is 95% silica—and when silica cools, it hardens.
This article presents the meaning, scope (boundaries) and the competence of engineering geology and geotechnics in historical, formal and practical (real) terms. Three groups of views are presented concerning the relation between engineering geology and geotechnics, their place and relationship against the background of other disciplines.
Geovisualisation is a developing field of computing science with the fundamental approach that displaying visual representations of data assists humans in generating ideas and hypotheses about the data set (e.g., [3, 4, 9]).In the applied sciences, coupling remote sensing and GIS-based mapping are helpful for data visualisation, spatial analysis, and a better understanding of the functioning ...
The engineering performance of soil after exposure to temperature is increasingly important in geotechnical and geoenvironmental engineering. Most thermal remediation of soil concentrates more on the … Expand
Geology-driven modeling: A new probabilistic approach for incorporating uncertain geological interpretations in 3D geological modeling. Rasmus Bødker Madsen, Anne-Sophie Høyer, Lærke Therese Andersen, Ingelise Møller, Thomas Mejer Hansen. Article 106833.
Copying or distributing in print or electronic forms without written permission of IGI Global is prohibited. Geophysical Surveys in Engineering Geology Investigations With Field Examples 1. INTRODUCTION This chapter focusses on geophysical survey techniques, employed in engineering geological investigations and it includes case studies.
The Journal of Geotechnical and Geoenvironmental Engineering covers the broad area of practice known as geotechnical engineering. Papers are welcomed on topics such as foundations, retaining structures, soil dynamics, engineering behavior of soil and rock, site characterization, slope stability, dams, rock engineering, earthquake engineering, environmental geotechnics, geosynthetics, computer ...
Geology. Geoscience. Landslides. ChapterPDF Available. Landslides: A Guide to Researching Landslide Phenomena and Processes. February 2015. DOI: 10.4018/978-1-4666-7336-6. In book: Handbook of ...
Published in Engineering Geology 1 May 2024; Geology, Engineering; View via Publisher. Save to Library Save. ... Showing 1 through 3 of 0 Related Papers. 17 References; Related Papers ... Semantic Scholar is a free, AI-powered research tool for scientific literature, based at the Allen Institute for AI. Learn More. About About Us Meet the Team ...
Evaluating innovative direct and indirect soil suction and volumetric measurement techniques for the determination of soil water retention curves following drying and wetting paths. Abdallah Najdi, David Encalada, Joao Mendes, Pere C. Prat, Alberto Ledesma. Article 107179.
The failure of rocks in seasonal frozen areas under freeze-thaw cycles (FTCs) is a frequently problem in engineering construction, posing a huge threat to the stability of the engineering. In order to explore the mechanism of rock damage degradation. It is necessary to analyze the damage evolution process of rocks and establish an accurate FTCs rock damage constitutive model. Taking the ...
This special issue of Natural Science, entitled Earthquakes, tackles a wide range of research subjects related to earthquakes, including their nature, measures, causes of occurrence, characteristics, attenuation modeling, soil wave amplification, methods and tools of recording, analysis and prediction, their effect on the built environment and methods of design, analysis, assessment, control ...