INFOGRAPHIC
Water distribution on earth.
Availability of freshwater is vital to live on Earth, yet freshwater makes up a tiny fraction of all the water available on the planet.
Ecology, Geology, Oceanography, Conservation
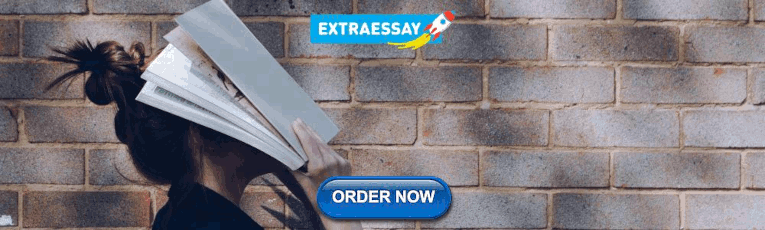
Loading ...
Although water covers about 70 percent of Earth's surface, only a small percentage of that water is freshwater, and even less of that is easily accessible to the billions of organisms that depend on freshwater for survival.
Media Credits
The audio, illustrations, photos, and videos are credited beneath the media asset, except for promotional images, which generally link to another page that contains the media credit. The Rights Holder for media is the person or group credited.
Last Updated
October 19, 2023
User Permissions
For information on user permissions, please read our Terms of Service. If you have questions about how to cite anything on our website in your project or classroom presentation, please contact your teacher. They will best know the preferred format. When you reach out to them, you will need the page title, URL, and the date you accessed the resource.
If a media asset is downloadable, a download button appears in the corner of the media viewer. If no button appears, you cannot download or save the media.
Text on this page is printable and can be used according to our Terms of Service .
Interactives
Any interactives on this page can only be played while you are visiting our website. You cannot download interactives.
Related Resources
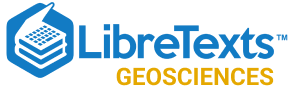
- school Campus Bookshelves
- menu_book Bookshelves
- perm_media Learning Objects
- login Login
- how_to_reg Request Instructor Account
- hub Instructor Commons
Margin Size
- Download Page (PDF)
- Download Full Book (PDF)
- Periodic Table
- Physics Constants
- Scientific Calculator
- Reference & Cite
- Tools expand_more
- Readability
selected template will load here
This action is not available.
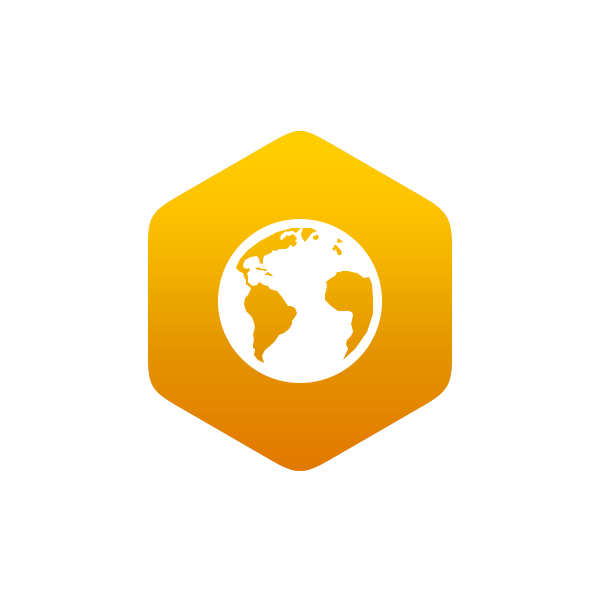
6.1: Distribution of Earth's Water
- Last updated
- Save as PDF
- Page ID 20783
\( \newcommand{\vecs}[1]{\overset { \scriptstyle \rightharpoonup} {\mathbf{#1}} } \)
\( \newcommand{\vecd}[1]{\overset{-\!-\!\rightharpoonup}{\vphantom{a}\smash {#1}}} \)
\( \newcommand{\id}{\mathrm{id}}\) \( \newcommand{\Span}{\mathrm{span}}\)
( \newcommand{\kernel}{\mathrm{null}\,}\) \( \newcommand{\range}{\mathrm{range}\,}\)
\( \newcommand{\RealPart}{\mathrm{Re}}\) \( \newcommand{\ImaginaryPart}{\mathrm{Im}}\)
\( \newcommand{\Argument}{\mathrm{Arg}}\) \( \newcommand{\norm}[1]{\| #1 \|}\)
\( \newcommand{\inner}[2]{\langle #1, #2 \rangle}\)
\( \newcommand{\Span}{\mathrm{span}}\)
\( \newcommand{\id}{\mathrm{id}}\)
\( \newcommand{\kernel}{\mathrm{null}\,}\)
\( \newcommand{\range}{\mathrm{range}\,}\)
\( \newcommand{\RealPart}{\mathrm{Re}}\)
\( \newcommand{\ImaginaryPart}{\mathrm{Im}}\)
\( \newcommand{\Argument}{\mathrm{Arg}}\)
\( \newcommand{\norm}[1]{\| #1 \|}\)
\( \newcommand{\Span}{\mathrm{span}}\) \( \newcommand{\AA}{\unicode[.8,0]{x212B}}\)
\( \newcommand{\vectorA}[1]{\vec{#1}} % arrow\)
\( \newcommand{\vectorAt}[1]{\vec{\text{#1}}} % arrow\)
\( \newcommand{\vectorB}[1]{\overset { \scriptstyle \rightharpoonup} {\mathbf{#1}} } \)
\( \newcommand{\vectorC}[1]{\textbf{#1}} \)
\( \newcommand{\vectorD}[1]{\overrightarrow{#1}} \)
\( \newcommand{\vectorDt}[1]{\overrightarrow{\text{#1}}} \)
\( \newcommand{\vectE}[1]{\overset{-\!-\!\rightharpoonup}{\vphantom{a}\smash{\mathbf {#1}}}} \)
Water is composed of two atoms of hydrogen and one atom of oxygen bonded together. Despite its simplicity, water has remarkable properties. Water expands when it freezes and has high surface tension because of the molecules’ polar nature that they tend to stick together. Without water, life might not exist on Earth, and it certainly would not have the tremendous complexity and diversity that we see.
Earth’s oceans contain 97 percent of the planet’s water, and just 3 percent is freshwater with relatively low concentrations of salts. Most freshwater is trapped as ice in the vast glaciers and ice sheets of Greenland and Antarctica. A storage location for water such as an ocean, glacier, pond, or even the atmosphere is known as a reservoir . A water molecule may pass through a reservoir very quickly or may remain for much longer. The amount of time a molecule stays in a reservoir is known as its residence time .
Hydrologic Cycle
Because of the unique properties of water, water molecules can cycle through almost any-where on Earth. The water molecule found in a glass of water today could have erupted from a volcano early in Earth history. In the intervening billions of years, the molecule probably spent time in a glacier or far below the ground. The molecule surely was high up in the atmosphere and maybe deep in the belly of a dinosaur.
Water is the only substance on Earth that is present in all three states of matter – as a solid, liquid, or gas. Along with that, Earth is the only planet where water is present in all three states. Because of the ranges in temperature in specific locations around the planet, all three phases may be present in a specific location or region. The three phases are solid (ice or snow), liquid (water), and gas (water vapor).

Water is continuously on the move. It is evaporated from the oceans, lakes, streams, the surface of the land, and plants ( transpiration ) by solar energy. It is moved through the atmosphere by winds and condenses to form clouds of water droplets or ice crystals. It comes back down as rain or snow and flows through streams, into lakes, and eventually back to the ocean. Water on the surface and in streams and lakes infiltrates the ground to become groundwater. Groundwater slowly moves through the rock and surficial materials. Some groundwater returns to other streams and lakes, and some go directly back to the oceans. (Earle, 2019)
Because Earth’s water is present in all three states, it can get into various environments around the planet. The movement of water around the Earth’s surface is the hydrologic (water) cycle. Water changes from a liquid to a gas by evaporation to become water vapor. The Sun’s energy can evaporate water from the ocean surface or lakes, streams, or puddles on land. Only the water molecules evaporate; the salts remain in the ocean or a freshwater reservoir. The water vapor stays in the atmosphere until it undergoes condensation to become tiny droplets of liquid. The droplets gather in clouds, which are blown about the globe by the wind. As the water droplets in the clouds collide and grow, they fall from the sky as precipitation. Precipitation can be rain, sleet, hail, or snow. Sometimes precipitation falls back into the ocean, and sometimes it falls onto the land surface.
When water falls from the sky as rain, it may enter streams and rivers that flow down to oceans and lakes. Water that falls as snow may sit on a mountain for several months. Snow may become part of the ice in a glacier, where it may remain for hundreds or thousands of years. Snow and ice may go directly back into the air by sublimation , the process in which a solid changes directly into a gas without first becoming a liquid. Although it is hard to see water vapor sublimate from a glacier, it is possible to see dry ice sublimate in the air.

A YouTube element has been excluded from this version of the text. You can view it online here: https://slcc.pressbooks.pub/physicalgeography/?p=509
Snow and ice slowly melt over time to become liquid water, which provides a steady flow of freshwater to streams, rivers, and lakes below. A water droplet falling as rain could also become part of a stream or a lake. At the surface, the water may eventually evaporate and reenter the atmosphere.

A significant amount of water infiltrates into the ground, and soil moisture is an important reservoir for that water. Water trapped in the soil is essential for plants to grow. Water may seep through dirt and rock below the soil through pores infiltrating the ground to go into Earth’s groundwater system. Groundwater may enter aquifers that may store freshwater for centuries. Alternatively, the water may come to the surface through springs or find its way back to the oceans. Plants and animals depend on water to live, and they also play a role in the water cycle. Plants take water from the soil and release substantial amounts of water vapor into the air through their leaves, a process known as transpiration. NASA has an excellent online animation of the hydrologic cycle.
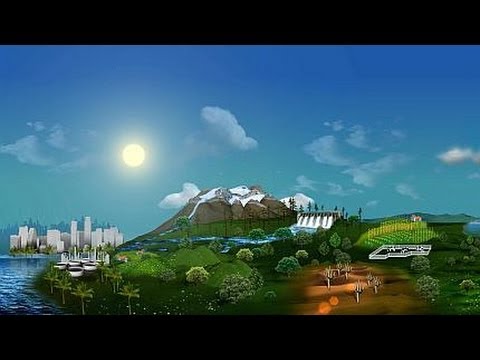
People also depend on water as a natural resource. Not content to get water directly from streams or ponds, humans create canals, aqueducts, dams, and wells to collect water and direct it to where they want it.
The table above displays water use in the United States and globally (Estimated Use of Water in the United States in 2005, USGS). It is important to note that water molecules cycle around. If climate cools and glaciers and ice caps grow, there is less water for the oceans, and sea level will fall. The reverse can also happen.
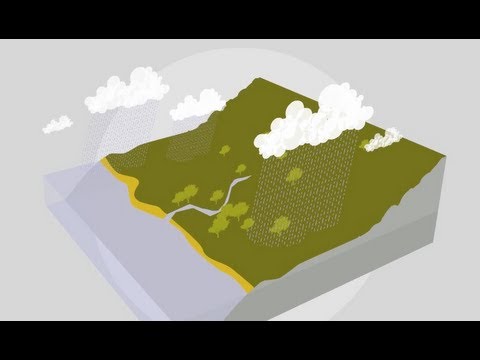
Earth’s Water Distribution
- Living reference work entry
- First Online: 09 September 2021
- Cite this living reference work entry
- Tarasova Oksana 7 &
- Gulevets Dmytro 8
Part of the book series: Encyclopedia of the UN Sustainable Development Goals ((ENUNSDG))
88 Accesses
Climate change ; Water demand ; Water quality ; Water use
Definitions
The Earth is a closed system, meaning that very little matter, including water, ever leaves or enters the atmosphere; the water that was here billions of years ago is still here now (NGWA; USGS).
Water means life on the Earth. Any living organism consists of water and its functioning is bound to water availability and water sources. Water on the Earth exists in solid, liquid, and gaseous forms always in constant redistribution process between these forms also known as the hydrologic cycle.
This cycle corresponds to the succession of stages through which water passes from the atmosphere to the Earth and returns to the atmosphere: evaporation from the land, sea, or inland water, condensation to form clouds, precipitation, interception, infiltration, percolation, runoff, accumulation in the soil or in bodies of water, and re-evaporation (NOAA, Water cycle).
Introduction
Water distribution.
The total volume of water...
This is a preview of subscription content, log in via an institution to check access.
Access this chapter
Institutional subscriptions
Allen GH, Pavelsky TM (2018) Global surface area of rivers and streams is 45 percent higher than previously thought. Science News. https://www.sciencedaily.com/releases/2018/06/180628151749.htm
Aral Sea. https://en.wikipedia.org/wiki/Aral_Sea
Babkin VI, EOLSS. Hydrological cycle – vol II – Evaporation from the surface of the globe – ©Encyclopedia of Life Support Systems (EOLSS). http://www.eolss.net/sample-chapters/c07/e2-02-03-02.pdf
Bar-On YM, Phillips R, Milo R (2018) The biomass distribution on Earth. Proc Natl Acad Sci USA 115(25):6506–6511. Bibcode:1998PNAS...95.6578W. https://doi.org/10.1073/pnas.1711842115
Article CAS Google Scholar
Britannica. Encyclopædia of Britannica, Inc. https://www.britannica.com/
Crandell CJ Wetland. https://www.britannica.com/science/wetland
Downing JA, Prairie YT et al (2006) The global abundance and size distribution of lakes, ponds, and impoundments. Limnol Oceanogr 51(5):2388–2397
Article Google Scholar
Eakins BW, Sharman GF (2010) Volumes of the world’s oceans from ETOPO1. NOAA National Geophysical Data Center, Boulder
Google Scholar
Falkenmark M, Rockstrom J (2004) Balancing water for humans and nature: the new approach in ecohydrology. Published in 2004 in London Sterling VA by Earthscan
FAO (2006) Concepts and definitions. http://www.fao.org/docrep/005/y4473e/y4473e06.htm
Freeze and Cherry (1979) Groundwater. http://hydrogeologistswithoutborders.org/wordpress/textbook-project/
GCOS (2004) Global climate observing system. https://library.wmo.int/doc_num.php?explnum_id=6348
Gleick PH (1993) Water in crisis: chapter 2. Oxford University Press. https://global.oup.com/ushe/product/water-in-crisis-9780195076288?cc=ua&lang=en&
Gleick PH (1996) Water resources. In: Encyclopedia of climate and weather, vol 2. Oxford University Press, New York, pp 817–823
GLOWABO. Global Lake and Wetlands Datbase. https://www.worldwildlife.org/pages/global-lakes-and-wetlands-database .
IGRAC. https://www.un-igrac.org/what-groundwater
IPCC (2007) Climate change 2007: the physical science basis. Intergovernmental Panel on Climate Change, Cambridge/New York. http://www.ipcc.ch/publications_and_data/ar4/syr/en/contents.html
Lake Baikal. https://en.wikipedia.org/wiki/Lake_Baikal
Lake Kariba. https://en.wikipedia.org/wiki/Lae_Kariba
Mekonnen MM, Hoekstra AY (2016) Four billion people facing severe water scarcity. Sci Adv 2(2):e1500323. https://doi.org/10.1126/sciadv.1500323
NGWA (2019) Distribution on Earth’s water. https://www.ngwa.org/what-is-groundwater/About-groundwater/information-on-earths-water
NOAA (2018) How many oceans are there? https://oceanservice.noaa.gov/facts/howmanyoceans.html
Osborn L Number of reservoirs in the world. Current Results, whether and science facts. https://www.currentresults.com/Environment-Facts/number-reservoirs-in-world.php
Pollard WH, French HM (1980) A first approximation of the volume of ground ice, RichardsIsland, Pleistocene Mackenzie Delta, Northwes tTerritories, Canada. Can Geotech J 17(4):509–516
River… http://www.scienceclarified.com/Qu-Ro/River.html
Salt lakes… https://en.wikipedia.org/wiki/Salt_lake
Shiklomanov I (1993) World freshwater resources. In: Gleick PH (ed) Water in crisis: a guide to the world’s freshwater resources. Oxford University Press, New York
Temple J (2017) How to pull water out of thin air, even in the driest parts of the globe. MIT Tech Rev. https://www.technologyreview.com/s/604137/water-from-desert-skies/
UNESCO Facts and figures http://www.unesco.org/new/en/natural-sciences/environment/water/wwap/facts-and-figures/all-facts-wwdr3/fact-14-ecosystems-river-regulation/
USGS (1984). The Fundamentals of the Water Cycle. https://www.usgs.gov/special-topic/water-science-school/science/fundamentals-water-cycle?qt-science_center_objects=0#qt-science_center_objects
USGS (2016). Transpiration – the water cycle, from USGS Water-Science School. https://water.usgs.gov/edu/watercycletranspiration.html
USGS (2017). https://pubs.usgs.gov/of/1993/ofr93-643/
Van Everdingen (1976) Multi-language glossary of permafrost and related ground-ice terms…The Arctic Institute of North America, the University of Calgary, Alberta (revised 2005)
Van Weert F, van der Gun J(2012) Saline and brackish groundwater at shallow and intermediate depths: genesis and world-wide occurrence. Presented during the IAH Congress in Canada. https://www.un-igrac.org/ru/resource/saline-and-brackish-groundwater-shallow-and-intermediate-depths-genesis-and-world-wide
Verpoorter C, Kutser T et al (2014) A global inventory of lakes based on high-resolution satellite imagery. Geophys Res Lett 41:6396–6402. https://doi.org/10.1002/2014GL060641
WGMS (2008) Global glacier change: facts and figures UNEP Global Monitoring Service Geneva/Zurich/Nairobi, 1 Sept 2008, pp 88
WRMS (2018) World register of marine species. http://www.marinespecies.org
Download references
Author information
Authors and affiliations.
National Ecological Center of Ukraine, Kyiv, Ukraine
Tarasova Oksana
Ecosafety Laboratory, National Aviation University, Kyiv, Ukraine
Gulevets Dmytro
You can also search for this author in PubMed Google Scholar
Editor information
Editors and affiliations.
European School of Sustainability, Hamburg University of Applied Sciences, Hamburg, Hamburg, Germany
Walter Leal Filho
Center for Neuroscience & Cell Biology, University of Coimbra, Coimbra, Portugal
Anabela Marisa Azul
Faculty of Engineering and Architecture, Passo Fundo University Faculty of Engineering and Architecture, Passo Fundo, Brazil
Luciana Brandli
HAW Hamburg, Hamburg, Hamburg, Germany
Amanda Lange Salvia
International Centre for Thriving, University of Chester, Chester, UK
Section Editor information
University of Aveiro, Aveiro, Portugal
Ulisses Azeiteiro
Rights and permissions
Reprints and permissions
Copyright information
© 2021 Springer Nature Switzerland AG
About this entry
Cite this entry.
Oksana, T., Dmytro, G. (2021). Earth’s Water Distribution. In: Leal Filho, W., Azul, A.M., Brandli, L., Lange Salvia, A., Wall, T. (eds) Clean Water and Sanitation. Encyclopedia of the UN Sustainable Development Goals. Springer, Cham. https://doi.org/10.1007/978-3-319-70061-8_103-1
Download citation
DOI : https://doi.org/10.1007/978-3-319-70061-8_103-1
Received : 17 July 2020
Accepted : 19 July 2020
Published : 09 September 2021
Publisher Name : Springer, Cham
Print ISBN : 978-3-319-70061-8
Online ISBN : 978-3-319-70061-8
eBook Packages : Springer Reference Earth and Environm. Science Reference Module Physical and Materials Science Reference Module Earth and Environmental Sciences
- Publish with us
Policies and ethics
- Find a journal
- Track your research
- Getting Help
- Online Student Library Guide
- Meet the Instructors
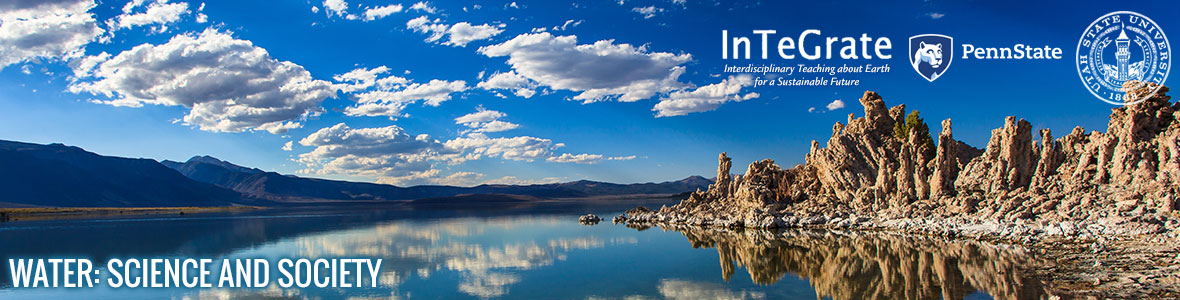
Water Distribution on Earth

Where is water distributed on Earth?
Earth is often called the “Blue Planet”, because of its abundance of liquid water. As we’ve already covered in Module #1, this water is distributed in the oceans, ice caps and glaciers, surface water (streams, lakes, and rivers), groundwater, soil moisture, the atmosphere, and in biomass. However, these reservoirs of Earth’s water are not static; water is constantly fluxing between them. We see this transport of water every day, for example in the form of flowing rivers, rain and snow, and groundwater springs.
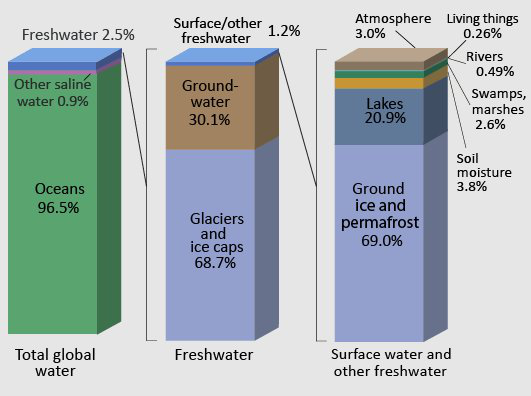
- Systems Thinking and the Hydrologic Cycle
- Hydrologic Cycle
- Uneven Distribution
- EO Explorer

- Global Maps
The Water Cycle
Viewed from space, one of the most striking features of our home planet is the water, in both liquid and frozen forms, that covers approximately 75% of the Earth’s surface. Geologic evidence suggests that large amounts of water have likely flowed on Earth for the past 3.8 billion years—most of its existence. Believed to have initially arrived on the surface through the emissions of ancient volcanoes, water is a vital substance that sets the Earth apart from the rest of the planets in our solar system. In particular, water appears to be a necessary ingredient for the development and nourishment of life.
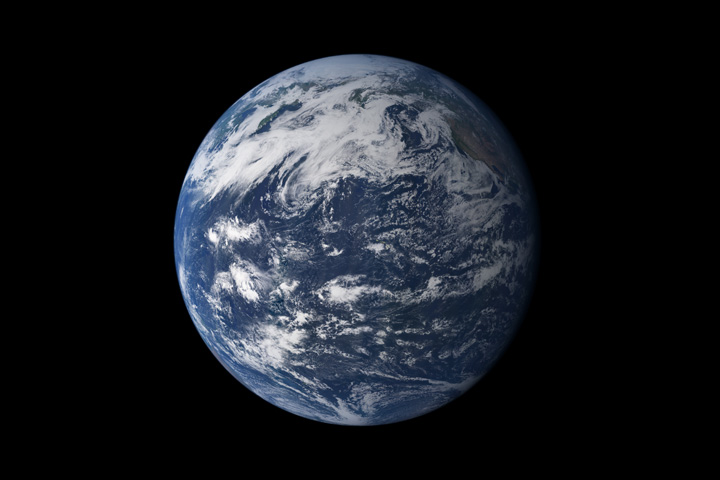
Earth is a water planet: three-quarters of the surface is covered by water, and water-rich clouds fill the sky. (NASA.)
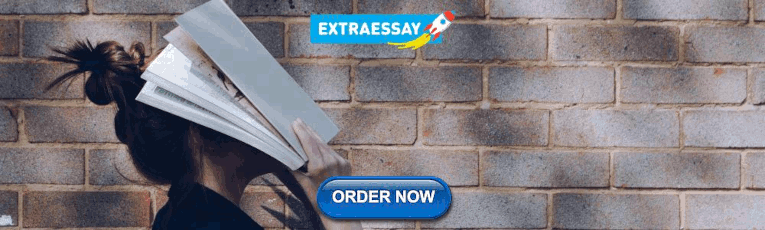
Water, Water, Everywhere
Water is practically everywhere on Earth. Moreover, it is the only known substance that can naturally exist as a gas, a liquid, and solid within the relatively small range of air temperatures and pressures found at the Earth’s surface.
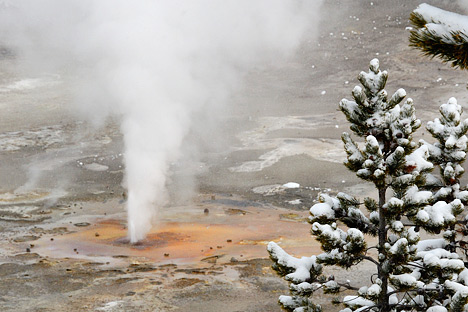
Water is the only common substance that can exist naturally as a gas, liquid, or solid at the relatively small range of temperatures and pressures found on the Earth’s surface. Sometimes, all three states are even present in the same time and place, such as this wintertime eruption of a geyser in Yellowstone National Park. (Photograph ©2008 haglundc. )
In all, the Earth’s water content is about 1.39 billion cubic kilometers (331 million cubic miles), with the bulk of it, about 96.5%, being in the global oceans. As for the rest, approximately 1.7% is stored in the polar icecaps, glaciers, and permanent snow, and another 1.7% is stored in groundwater, lakes, rivers, streams, and soil. Only a thousandth of 1% of the water on Earth exists as water vapor in the atmosphere.
Despite its small amount, this water vapor has a huge influence on the planet. Water vapor is a powerful greenhouse gas, and it is a major driver of the Earth’s weather and climate as it travels around the globe, transporting latent heat with it. Latent heat is heat obtained by water molecules as they transition from liquid or solid to vapor; the heat is released when the molecules condense from vapor back to liquid or solid form, creating cloud droplets and various forms of precipitation.
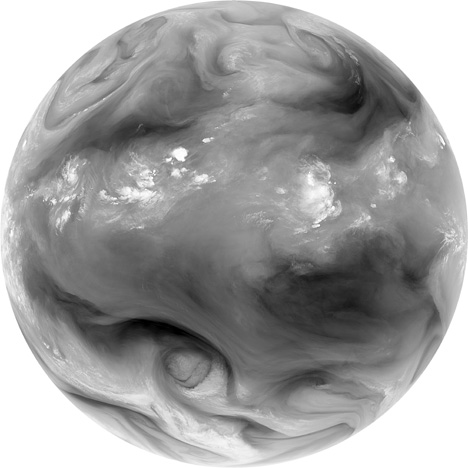
Water vapor—and with it energy—is carried around the globe by weather systems. This satellite image shows the distribution of water vapor over Africa and the Atlantic Ocean. White areas have high concentrations of water vapor, while dark regions are relatively dry. The brightest white areas are towering thunderclouds. The image was acquired on the morning of September 2, 2010 by SEVIRI aboard METEOSAT-9. [Watch this animation (23 MB QuickTime) of similar data to see the movement of water vapor over time.] (Image ©2010 EUMETSAT. )
For human needs, the amount of freshwater on Earth—for drinking and agriculture—is particularly important. Freshwater exists in lakes, rivers, groundwater, and frozen as snow and ice. Estimates of groundwater are particularly difficult to make, and they vary widely. (The value in the above table is near the high end of the range.)
Groundwater may constitute anywhere from approximately 22 to 30% of fresh water, with ice (including ice caps, glaciers, permanent snow, ground ice, and permafrost) accounting for most of the remaining 78 to 70%.
A Multi-Phased Journey
The water, or hydrologic, cycle describes the pilgrimage of water as water molecules make their way from the Earth’s surface to the atmosphere and back again, in some cases to below the surface. This gigantic system, powered by energy from the Sun, is a continuous exchange of moisture between the oceans, the atmosphere, and the land.
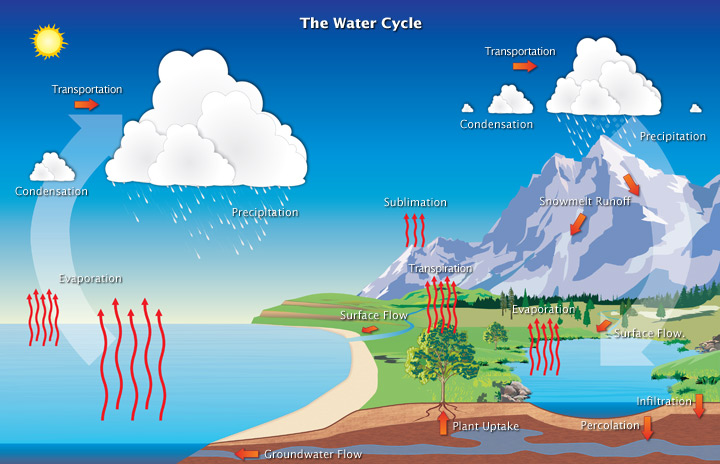
Earth’s water continuously moves through the atmosphere, into and out of the oceans, over the land surface, and underground. ( Image courtesy NOAA National Weather Service Jetstream. )
Studies have revealed that evaporation—the process by which water changes from a liquid to a gas—from oceans, seas, and other bodies of water (lakes, rivers, streams) provides nearly 90% of the moisture in our atmosphere. Most of the remaining 10% found in the atmosphere is released by plants through transpiration. Plants take in water through their roots, then release it through small pores on the underside of their leaves. In addition, a very small portion of water vapor enters the atmosphere through sublimation, the process by which water changes directly from a solid (ice or snow) to a gas. The gradual shrinking of snow banks in cases when the temperature remains below freezing results from sublimation.
Together, evaporation, transpiration, and sublimation, plus volcanic emissions, account for almost all the water vapor in the atmosphere that isn’t inserted through human activities. While evaporation from the oceans is the primary vehicle for driving the surface-to-atmosphere portion of the hydrologic cycle, transpiration is also significant. For example, a cornfield 1 acre in size can transpire as much as 4,000 gallons of water every day.
After the water enters the lower atmosphere, rising air currents carry it upward, often high into the atmosphere, where the air is cooler. In the cool air, water vapor is more likely to condense from a gas to a liquid to form cloud droplets. Cloud droplets can grow and produce precipitation (including rain, snow, sleet, freezing rain, and hail), which is the primary mechanism for transporting water from the atmosphere back to the Earth’s surface.
When precipitation falls over the land surface, it follows various routes in its subsequent paths. Some of it evaporates, returning to the atmosphere; some seeps into the ground as soil moisture or groundwater; and some runs off into rivers and streams. Almost all of the water eventually flows into the oceans or other bodies of water, where the cycle continues. At different stages of the cycle, some of the water is intercepted by humans or other life forms for drinking, washing, irrigating, and a large variety of other uses.
Groundwater is found in two broadly defined layers of the soil, the “zone of aeration,” where gaps in the soil are filled with both air and water, and, further down, the “zone of saturation,” where the gaps are completely filled with water. The boundary between these two zones is known as the water table, which rises or falls as the amount of groundwater changes.
The amount of water in the atmosphere at any moment in time is only 12,900 cubic kilometers, a minute fraction of Earth’s total water supply: if it were to completely rain out, atmospheric moisture would cover the Earth’s surface to a depth of only 2.5 centimeters. However, far more water—in fact, some 495,000 cubic kilometers of it—are cycled through the atmosphere every year. It is as if the entire amount of water in the air were removed and replenished nearly 40 times a year.
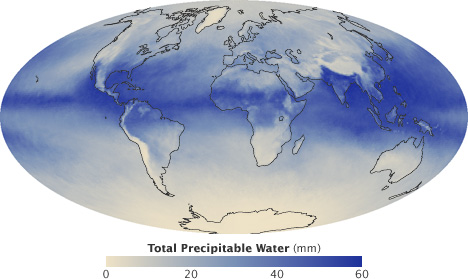
This map shows the distribution of water vapor throughout the depth of the atmosphere during August 2010. Even the wettest regions would form a layer of water only 60 millimeters deep if it were condensed at the surface. (NASA image by Robert Simmon, using AIRS & AMSU data.)
Water continually evaporates, condenses, and precipitates, and on a global basis, evaporation approximately equals precipitation. Because of this equality, the total amount of water vapor in the atmosphere remains approximately the same over time. However, over the continents, precipitation routinely exceeds evaporation, and conversely, over the oceans, evaporation exceeds precipitation.
In the case of the oceans, the continual excess of evaporation versus precipitation would eventually leave the oceans empty if they were not being replenished by additional means. Not only are they being replenished, largely through runoff from the land areas, but over the past 100 years, they have been over- replenished: sea level around the globe has risen approximately 17 centimeters over the course of the twentieth century.
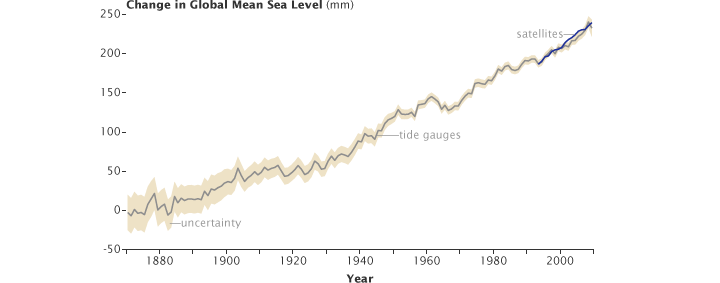
Sea level has been rising over the past century, partly due to thermal expansion of the ocean as it warms, and partly due to the melting of glaciers and ice caps. (Graph ©2010 Australian Commonwealth Scientific and Research Organization. )
Sea level has risen both because of warming of the oceans, causing water to expand and increase in volume, and because more water has been entering the ocean than the amount leaving it through evaporation or other means. A primary cause for increased mass of water entering the ocean is the calving or melting of land ice (ice sheets and glaciers). Sea ice is already in the ocean, so increases or decreases in the annual amount of sea ice do not significantly affect sea level.
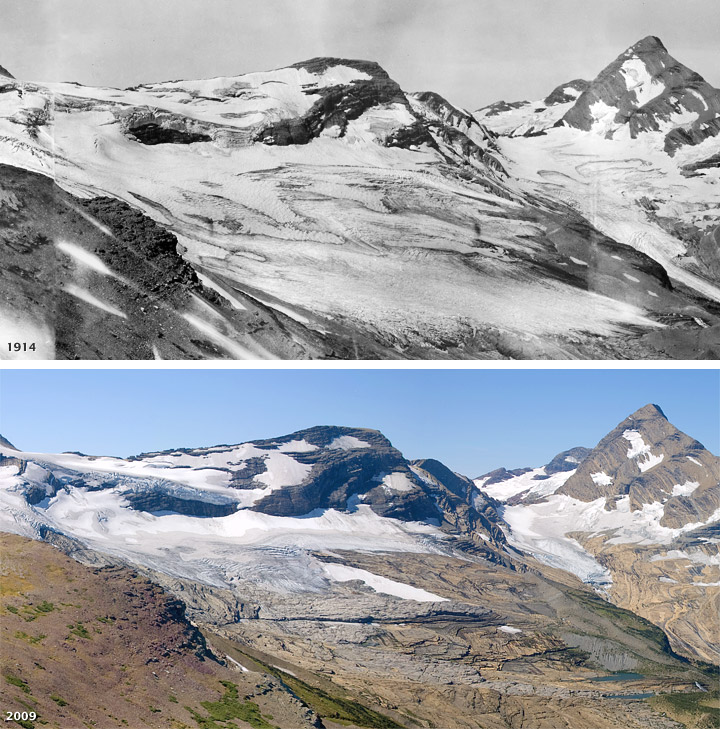
Blackfoot (left) and Jackson (right) glaciers, both in the mountains of Glacier National Park, were joined along their margins in 1914, but have since retreated into separate alpine cirques. The melting of glacial ice is a major contributor to sea level rise. [ Photographs by E. B. Stebinger, Glacier National Park archives (1911), and Lisa McKeon, USGS (2009).]
Throughout the hydrologic cycle, there are many paths that a water molecule might follow. Water at the bottom of Lake Superior may eventually rise into the atmosphere and fall as rain in Massachusetts. Runoff from the Massachusetts rain may drain into the Atlantic Ocean and circulate northeastward toward Iceland, destined to become part of a floe of sea ice, or, after evaporation to the atmosphere and precipitation as snow, part of a glacier.
Water molecules can take an immense variety of routes and branching trails that lead them again and again through the three phases of ice, liquid water, and water vapor. For instance, the water molecules that once fell 100 years ago as rain on your great- grandparents’ farmhouse in Iowa might now be falling as snow on your driveway in California.
The Water Cycle and Climate Change
Among the most serious Earth science and environmental policy issues confronting society are the potential changes in the Earth’s water cycle due to climate change. The science community now generally agrees that the Earth’s climate is undergoing changes in response to natural variability, including solar variability, and increasing concentrations of greenhouse gases and aerosols. Furthermore, agreement is widespread that these changes may profoundly affect atmospheric water vapor concentrations, clouds, precipitation patterns, and runoff and stream flow patterns.
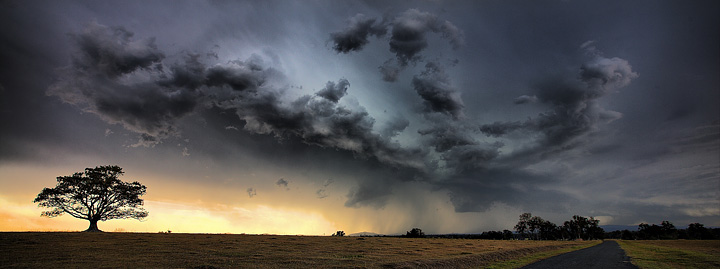
Global climate change will affect the water cycle, likely creating perennial droughts in some areas and frequent floods in others. ( Photograph ©2008 Garry Schlatter. )
For example, as the lower atmosphere becomes warmer, evaporation rates will increase, resulting in an increase in the amount of moisture circulating throughout the troposphere (lower atmosphere). An observed consequence of higher water vapor concentrations is the increased frequency of intense precipitation events, mainly over land areas. Furthermore, because of warmer temperatures, more precipitation is falling as rain rather than snow.
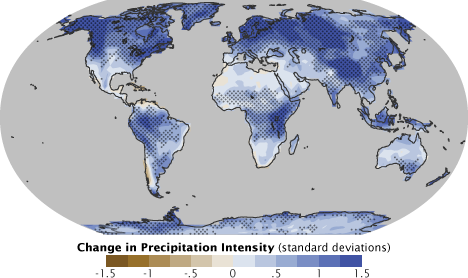
One expected effect of climate change will be an increase in precipitation intensity: a larger proportion of rain will fall in a shorter amount of time than it has historically. Blue represents areas where climate models predict an increase in intensity by the end of the 21st century, brown represents a predicted decrease. (Map adapted from the IPCC Fourth Assessment Report.)
In parts of the Northern Hemisphere, an earlier arrival of spring-like conditions is leading to earlier peaks in snowmelt and resulting river flows. As a consequence, seasons with the highest water demand, typically summer and fall, are being impacted by a reduced availability of fresh water.
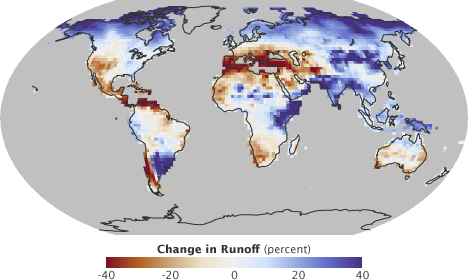
Changes in water runoff into rivers and streams are another expected consequence of climate change by the late 21st Century. This map shows predicted increases in runoff in blue, and decreases in brown and red. (Map by Robert Simmon, using data from Chris Milly, NOAA Geophysical Fluid Dynamics Laboratory.)
Warmer temperatures have led to increased drying of the land surface in some areas, with the effect of an increased incidence and severity of drought. The Palmer Drought Severity Index, which is a measure of soil moisture using precipitation measurements and rough estimates of changes in evaporation, has shown that from 1900 to 2002, the Sahel region of Africa has been experiencing harsher drought conditions. This same index also indicates an opposite trend in southern South America and the south central United States.
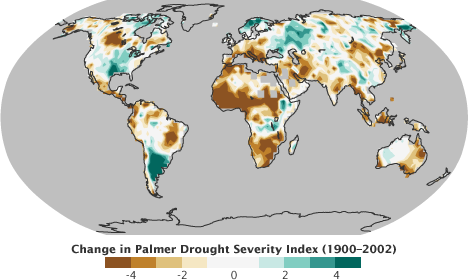
Shifts in the water cycle occurred over the past century due to a combination of natural variations and human forcings. From 1900 to 2002, droughts worsened in Sub-Saharan and southern Africa, eastern Brazil, and Iran (brown). Over the same period western Russia, south-eastern South America, Scandinavia, and the southern United States had less severe droughts (green). (Map adapted from the IPCC Fourth Assessment Report.)
While the brief scenarios described above represent a small portion of the observed changes in the water cycle, it should be noted that many uncertainties remain in the prediction of future climate. These uncertainties derive from the sheer complexity of the climate system, insufficient and incomplete data sets, and inconsistent results given by current climate models. However, state of the art (but still incomplete and imperfect) climate models do consistently predict that precipitation will become more variable, with increased risks of drought and floods at different times and places.
Observing the Water Cycle
Orbiting satellites are now collecting data relevant to all aspects of the hydrologic cycle, including evaporation, transpiration, condensation, precipitation, and runoff. NASA even has one satellite, Aqua, named specifically for the information it is collecting about the many components of the water cycle.
Aqua launched on May 4, 2002, with six Earth-observing instruments: the Atmospheric Infrared Sounder (AIRS), the Advanced Microwave Sounding Unit (AMSU), the Humidity Sounder for Brazil (HSB), the Advanced Microwave Scanning Radiometer for the Earth Observing System (AMSR-E), the Moderate Resolution Imaging Spectroradiometer (MODIS), and Clouds and the Earth’s Radiant Energy System (CERES).
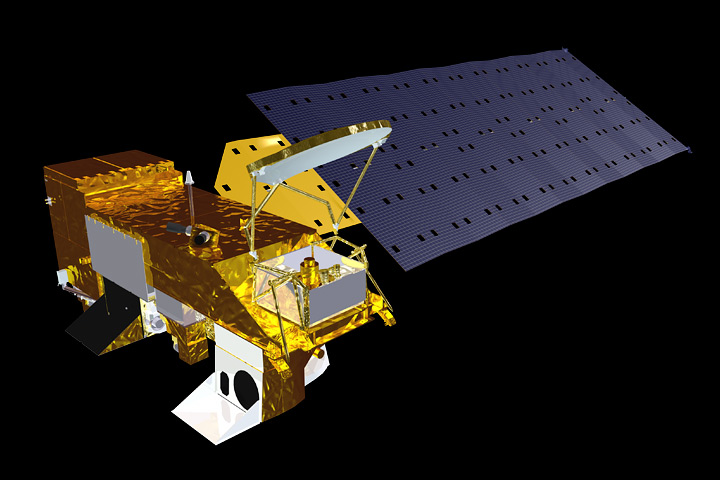
NASA’s Aqua satellite carries a suite of instruments designed primarily to study the water cycle. (NASA image by Marit Jentoft-Nilsen.)
Since water vapor is the Earth’s primary greenhouse gas, and it contributes significantly to uncertainties in projections of future global warming, it is critical to understand how it varies in the Earth system. In the first years of the Aqua mission, AIRS, AMSU, and HSB provided space-based measurements of atmospheric temperature and water vapor that were more accurate than any obtained before; the sensors also made measurements from more altitudes than any previous sensor. The HSB is no longer operational, but the AIRS/AMSU system continues to provide high-quality atmospheric temperature and water vapor measurements.
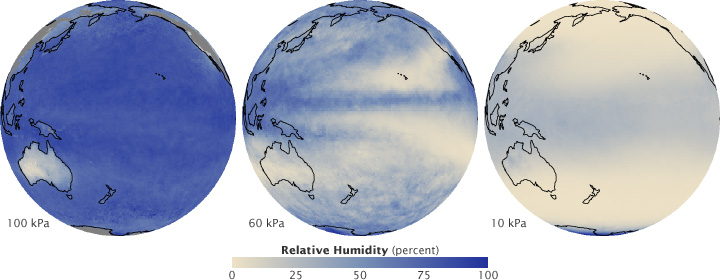
Aqua’s AIRS and AMSU instruments measure relative humidity at multiple pressure levels, which correspond to altitude. Near the surface (100 kPa), the air above the ocean is almost saturated with water, while it is dry above Australia. It is generally drier higher in the atmosphere (60 kPa), except where convection lifts moisture aloft. At the lower edge of the stratosphere (10 kPa) the air is almost universally dry. (NASA maps by Robert Simmon, based on AIRS/AMSU data.)
More recent studies using AIRS data have demonstrated that most of the warming caused by carbon dioxide does not come directly from carbon dioxide, but rather from increased water vapor and other factors that amplify the initial warming. Other studies have shown improved estimation of the landfall of a hurricane in the Bay of Bengal by incorporating AIRS temperature measurements, and improved understanding of large-scale atmospheric patterns such as the Madden-Julian Oscillation.
In addition to their importance to our weather, clouds play a major role in regulating Earth’s climate system. MODIS, CERES, and AIRS all collect data relevant to the study of clouds. The cloud data include the height and area of clouds, the liquid water they contain, and the sizes of cloud droplets and ice particles. The size of cloud particles affects how they reflect and absorb incoming sunlight, and the reflectivity (albedo) of clouds plays a major role in Earth’s energy balance.
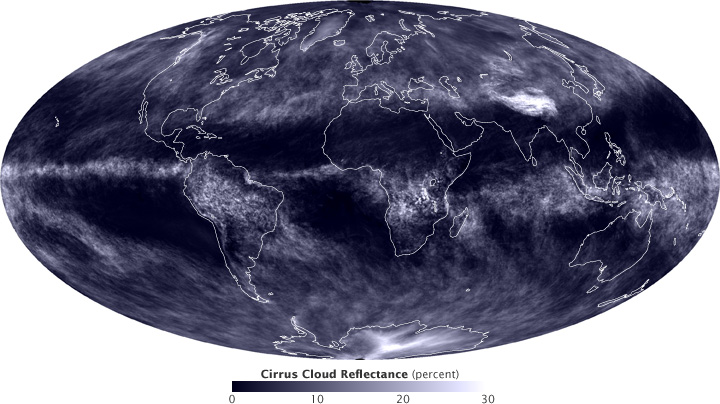
High, thin cirrus clouds reflect relatively little sunlight back into space compared to the amount reflected by thick cumulus clouds. This map shows the reflectivity of cirrus clouds [with a maximum of 30 percent (shown in white)] during March of 2010. (Map by Robert Simmon, using data from the MODIS Atmosphere Team. )
One of the many variables AMSR-E monitors is global precipitation. The sensor measures microwave energy, some of which passes through clouds, and so the sensor can detect the rainfall even under the clouds.
Water in the atmosphere is hardly the only focus of the Aqua mission. Among much else, AMSR-E and MODIS are being used to study sea ice. Sea ice is important to the Earth system not just as an important element in the habitat of polar bears, penguins, and some species of seals, but also because it can insulate the underlying liquid water against heat loss to the often frigid overlying polar atmosphere and because it reflects sunlight that would otherwise be available to warm the ocean.
When it comes to sea ice, AMSR-E and MODIS provide complementary information. AMSR-E doesn’t record as much detail about ice features as MODIS does, but it can distinguish ice versus open water even when it is cloudy. The AMSR-E measurements continue, with improved resolution and accuracy, a satellite record of changes in the extent of polar ice that extends back to the 1970s.
AMSR-E and MODIS also provide monitoring of snow coverage over land, another key indicator of climate change. As with sea ice, AMSR-E allows routine monitoring of the snow, irrespective of cloud cover, but with less spatial detail, while MODIS sees greater spatial detail, but only under cloud-free conditions.
As for liquid water on land, AMSR-E provides information about soil moisture, which is crucial for vegetation including agricultural crops. AMSR-E’s monitoring of soil moisture globally permits, for example, the early identification of signs of drought.
More Water Cycle Observations
Aqua is the most comprehensive of NASA’s water cycle missions, but it isn’t alone. In fact, the Terra satellite also has MODIS and CERES instruments onboard, and several other spacecraft have made or are making unique water-cycle measurements.
The Ice, Cloud, and Land Elevation Satellite (ICESat) was launched in January 2003, and it collected data on the topography of the Earth’s ice sheets, clouds, vegetation, and the thickness of sea ice off and on until October 2009. A new ICESat mission, ICESat-2, is now under development and is scheduled to launch in 2015.
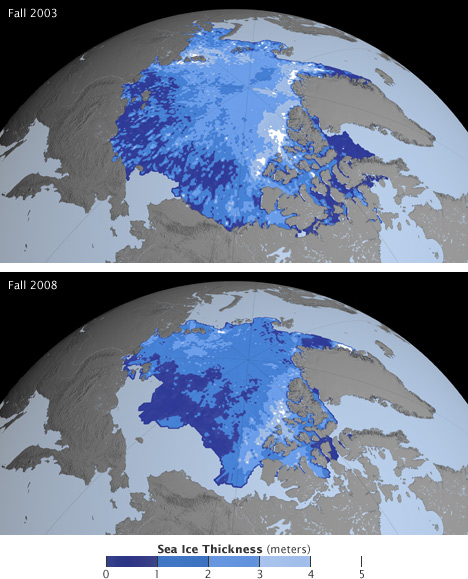
ICESat’s precise observation of the surface elevation of Arctic sea ice enabled measurement of ice thickness. These images show that sea ice thinned from fall 2003 to fall 2008. Dark blue areas are thin ice, white areas are thick ice, gray regions are land, and light blue south of the ice pack represents open water. (NASA images by the NASA GSFC Scientific Visualization Studio, using ICESat data.)
The Gravity Recovery and Climate Experiment (GRACE) is a unique mission that consists of two spacecraft orbiting one behind the other; changes in the distance between the two provide information about the gravity field on the Earth below. Because gravity depends on mass, some of the changes in gravity over time signal a shift in water from one place on Earth to another. Through measurements of changing gravity fields, GRACE scientists are able to derive information about changes in the mass of ice sheets and glaciers and even changes in groundwater around the world.
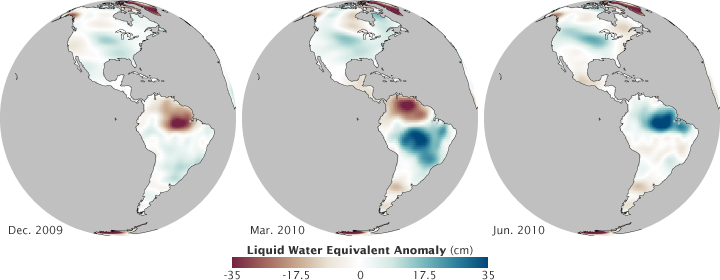
These GRACE data show monthly gravity differences calculated from a 2003-2007 baseline. The big contrasts in the Amazon are due to seasonal changes in rainfall. (NASA maps by Robert Simmon, using GRACE data. )
CloudSat is advancing scientists’ understanding of cloud abundance, distribution, structure, and radiative properties (how they absorb and emit energy, including thermal infrared energy escaping from Earth’s surface). Since 2006, CloudSat has flown the first satellite-based, millimeter-wavelength cloud radar—an instrument that is 1000 times more sensitive than existing weather radars on the ground. Unlike ground-based weather radars that use centimeter wavelengths to detect raindrop-sized particles, CloudSat’s radar allows the detection of the much smaller particles of liquid water and ice in the large cloud masses that contribute significantly to our weather.
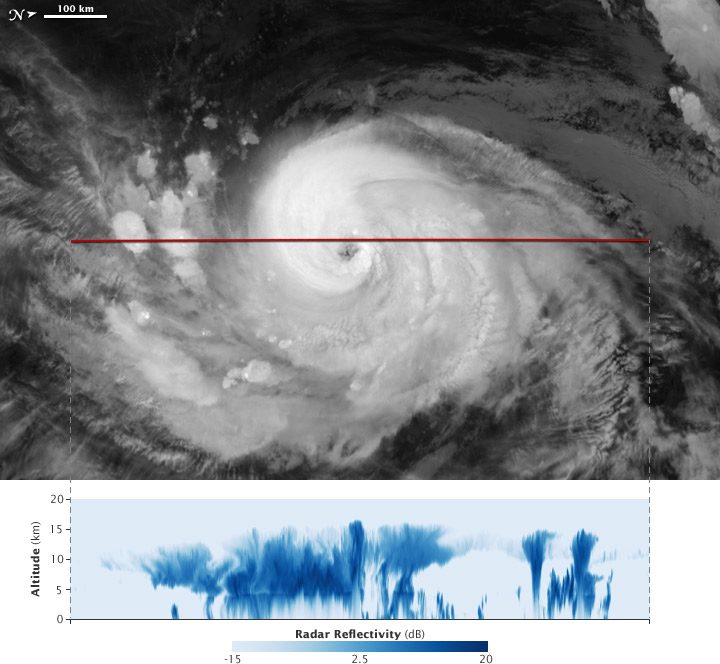
CloudSat’s radar measures the vertical distribution of clouds, such as this profile of Hurricane Julia. (NASA image by Jesse Allen, based on MODIS and CloudSat data.)
The joint NASA and French Cloud-Aerosol Lidar and Infrared Pathfinder Satellite Observations (CALIPSO) is providing new insight into the role that clouds and atmospheric aerosols (particles like dust and pollution) play in regulating Earth’s weather, climate, and air quality. CALIPSO combines an active laser instrument with passive infrared and visible imagers to probe the vertical structure and properties of thin clouds and aerosols over the globe.
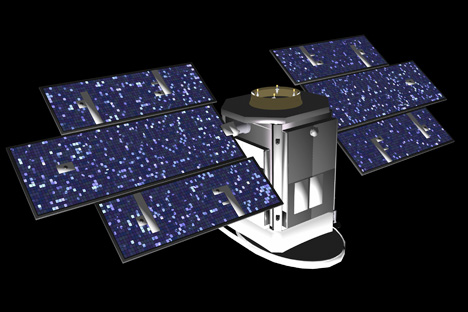
CloudSat (top) and CALIPSO (lower) are two satellites providing detailed views of the structure of clouds. (NASA images by Marit Jentoft-Nilsen.)
- Ahrens, C. (1994). Meteorology: An Introduction to Weather, Climate, and the Environment. St. Paul: West Publishing Company.
- Chahine, M. (1992). The hydrological cycle and its influence on climate. Nature, 359, 373-380.
- Lutgens, F., and Tarbuck, E. (1998). The Atmosphere: An Introduction to Meteorology. Upper Saddle River: Prentice Hall.
- Kundzewicz, Z. W., Mata, L. J., Arnell, N. W., Döll, P., Kabat, P., Jiménez, B., Miller, K. A., et al. (2007). Freshwater resources and their management. In M.L. Parry, O.F. Canziani, J.P. Palutikof, P.J. van der Linden, and C.E. Hanson, (Eds.), Climate Change 2007: Impacts, Adaptation, and Vulnerability. Contribution of Working Group II to the Fourth Assessment Report of the Intergovernmental Panel on Climate Change (173–210). Cambridge: Cambridge University Press.
- Moran, J., and Morgan, M. (1997). Meteorology: The Atmosphere and the Science of Weather. Upper Saddle River: Prentice Hall.
- Parkinson, C. L. (2010). Coming Climate Crisis? Consider the Past, Beware the Big Fix. Lanham, Maryland: Rowman & Littlefield Publishers.
- Rosenzweig, C., Casassa, G., Karoly, D.J., Imeson, A., Liu, C., Menzel, A., Rawlins, A., Root, T.L., Seguin, B., and Tryjanowski, P. (2007). Assessment of observed changes and responses in natural and managed systems. In M.L. Parry, O.F. Canziani, J.P. Palutikof, P.J. van der Linden, and C.E. Hanson, (Eds.), Climate Change 2007: Impacts, Adaptation, and Vulnerability. Contribution of Working Group II to the Fourth Assessment Report of the Intergovernmental Panel on Climate Change (79-131). Cambridge: Cambridge University Press.
- Schneider, S. (1996). Encyclopedia of Climate and Weather. New York: Oxford University Press.
Atmosphere Land Life Water
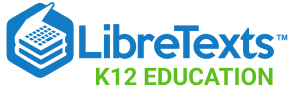
- school Campus Bookshelves
- menu_book Bookshelves
- perm_media Learning Objects
- login Login
- how_to_reg Request Instructor Account
- hub Instructor Commons
Margin Size
- Download Page (PDF)
- Download Full Book (PDF)
- Periodic Table
- Physics Constants
- Scientific Calculator
- Reference & Cite
- Tools expand_more
- Readability
selected template will load here
This action is not available.
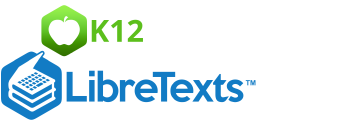
8.5: Water Distribution
- Last updated
- Save as PDF
- Page ID 5443
\( \newcommand{\vecs}[1]{\overset { \scriptstyle \rightharpoonup} {\mathbf{#1}} } \)
\( \newcommand{\vecd}[1]{\overset{-\!-\!\rightharpoonup}{\vphantom{a}\smash {#1}}} \)
\( \newcommand{\id}{\mathrm{id}}\) \( \newcommand{\Span}{\mathrm{span}}\)
( \newcommand{\kernel}{\mathrm{null}\,}\) \( \newcommand{\range}{\mathrm{range}\,}\)
\( \newcommand{\RealPart}{\mathrm{Re}}\) \( \newcommand{\ImaginaryPart}{\mathrm{Im}}\)
\( \newcommand{\Argument}{\mathrm{Arg}}\) \( \newcommand{\norm}[1]{\| #1 \|}\)
\( \newcommand{\inner}[2]{\langle #1, #2 \rangle}\)
\( \newcommand{\Span}{\mathrm{span}}\)
\( \newcommand{\id}{\mathrm{id}}\)
\( \newcommand{\kernel}{\mathrm{null}\,}\)
\( \newcommand{\range}{\mathrm{range}\,}\)
\( \newcommand{\RealPart}{\mathrm{Re}}\)
\( \newcommand{\ImaginaryPart}{\mathrm{Im}}\)
\( \newcommand{\Argument}{\mathrm{Arg}}\)
\( \newcommand{\norm}[1]{\| #1 \|}\)
\( \newcommand{\Span}{\mathrm{span}}\) \( \newcommand{\AA}{\unicode[.8,0]{x212B}}\)
\( \newcommand{\vectorA}[1]{\vec{#1}} % arrow\)
\( \newcommand{\vectorAt}[1]{\vec{\text{#1}}} % arrow\)
\( \newcommand{\vectorB}[1]{\overset { \scriptstyle \rightharpoonup} {\mathbf{#1}} } \)
\( \newcommand{\vectorC}[1]{\textbf{#1}} \)
\( \newcommand{\vectorD}[1]{\overrightarrow{#1}} \)
\( \newcommand{\vectorDt}[1]{\overrightarrow{\text{#1}}} \)
\( \newcommand{\vectE}[1]{\overset{-\!-\!\rightharpoonup}{\vphantom{a}\smash{\mathbf {#1}}}} \)
What do you have to do to get water?
In the developed world, we just open a tap. Clean water is available all the time. In many developing regions, people often have to walk long distances to get clean water. Sometimes there isn't a source of clean water, and they just get what they can find. We take water for granted in the developed world.
Water Problems: Not Enough Water
Most Americans have plenty of fresh, clean water, but many people around the world do not. In fact, water scarcity is the world’s most serious resource problem. How can that be? Water is almost everywhere, but much of it is unusable. What is usable is not always where it is needed.
Where Is All The Water?
One problem is that only a tiny fraction of Earth’s water is fresh, liquid water that people can use. More than 97 percent of Earth’s water is salt water in the oceans. Just 3% is freshwater. Most of the freshwater is frozen in ice sheets, icebergs, and glaciers ( Figure below).
This glacier in Alaska stores a lot of frozen freshwater.
Rainfall and Water Supply
Rainfall varies around the globe. About 40% of the world's landmass gets very little rain. About the same percentage of the world’s people don’t have enough water. You can compare global rainfall with the worldwide freshwater supply at the two URLs below. Drier climates generally have less water for people to use. In some places, people may have less water available to them for an entire year than many Americans use in a single day! How much water is there where you live?
Wealth and the Water Supply
Richer nations can drill deep wells, build large dams, or supply people with water in other ways. In these countries, just about everyone has access to clean running water in their homes, and water is cheap and easy to get. It’s no surprise that people in these countries use the most water. In poorer nations, there is little money to develop water supplies. Therefore, people get their water where they can.
Water Shortages
Water shortages are common in much of the world. People are most likely to run short of water during droughts. A drought is a period of unusually low rainfall. Human actions have increased how often droughts occur. One way people exacerbate droughts is by cutting down trees. Trees add a lot of water vapor to the air. With fewer trees, the air is drier and droughts are more common.
We already use six times as much water today as we did a hundred years ago. As the number of people rises, our need for water will grow. By the year 2025, only half the world’s people will have enough clean water. Water is such a vital resource that serious water shortages may cause other problems.
- Crops and livestock may die, so people will have less food available.
- Other uses of water, such as industry, may have to stop. This reduces the jobs people can get and the products they can buy.
- People and nations may fight over water resources.
- In extreme cases, people may die from lack of water.
Figure below shows the global water situation in the 2030s with water stress and water scarcity on the map.
Blues indicate no predicted water stress; pinks and lavenders predict water stress, and salmon to brown indicates water scarcity (variations of those colors indicate the amount of irrigation that will be done to produce crops).
- Fresh water is not evenly distributed across the planet.
- Many of the world's people live without access to enough clean water. That percentage is likely to increase as populations grow.
- During a drought, life may become very hard for many people.
- How is Earth's water distributed?
- How do wealthier nations deal with water problems? How do poorer nations deal with water problems?
- What is a drought? What can happen during a drought?
Explore More
Use the resource below to answer the questions that follow.
- What is water scarcity?
- Why do people take water for granted?
- How much freshwater is there on Earth?
- How many people do not have access to clean water?
- What will occur by 2025?
- What is physical water scarcity? Where does this occur?
- What is economic water scarcity? Where does this occur?
- What are two things you can do to reduce your water consumption?
NOTIFICATIONS
Earth’s water distribution.
- + Create new collection
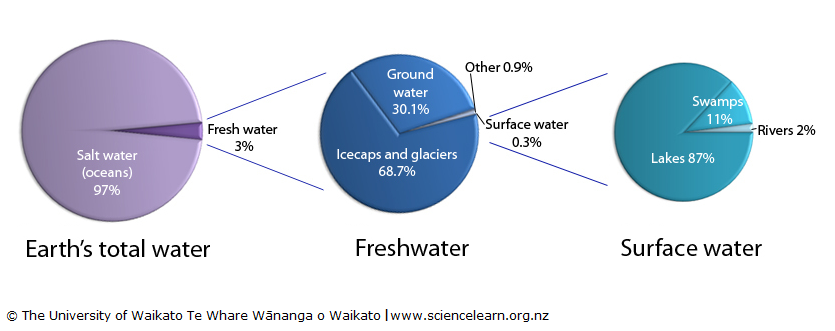
Over 70% of the Earth’s surface is covered in water, yet most of this is made up of seawater in oceans. Just a small amount is freshwater.

Water origins
Water contains atoms of oxygen and hydrogen, and life on Earth would not have evolved without it. Fruit and vegetables are made of 80% water, and our bodies are 60–70% water. (We lose about 2.5 ...
See our newsletters here .
Would you like to take a short survey?
This survey will open in a new tab and you can fill it out after your visit to the site.
Warning: Your Internet Explorer is out of date
Upgrade now to patch security holes.
You are using Internet Explorer 6 (IE6), which was released on August 27, 2001. Microsoft has discontinued support for IE6—as have many websites, including this one. Many websites no longer function properly in IE6. Additionally, IE6 has known security vulnerabilities that may allow attackers to take over your computer and steal your personal data.
Upgrading is easy
There's an easy solution: download and install a free, up-to-date browser. We strongly recommend any of the following:

Warning: JavaScript is not enabled in your browser
Some parts of this site won't work without JavaScript. Don't worry: It's easy to fix. Learn how to enable JavaScript in your browser
- Graphing Water on Earth
Implementation Options
Understanding student responses, preview and assign.

What is this task about?
- DCI: ESS2.C: The roles of water in Earth’s surface processes
- SEP: Using Mathematics and Computational Thinking
- CCC: Scale, Proportion, and Quantity
When To Use
Pick a paired task .
- Maya Moves to Los Angeles
- Water Supply from Shasta Lake
- Water Storage in Shasta Lake
- A Drop of Fresh Water and an Ocean of Salt Water
- Singapore's Four National Taps
Related Tasks

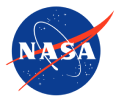
28 min read
Summary of the 2023 GRACE Follow-On Science Team Meeting

Felix Landerer , NASA/Jet Propulsion Laboratory, [email protected]
Introduction
In October 2023, the annual gathering of the Gravity Recovery and Climate Experiment (GRACE) and GRACE Follow-On [G-FO] Science Team took place in Boulder, CO, hosted at University Corporation for Atmospheric Research’s (UCAR) Center Green campus. The event had 70 in-person participant and an additional 52 online participants – see Photo . G-FO is a U.S.–German collaboration between NASA and the Helmholtz Centre Potsdam GeoForschungsZentrum (GFZ) [German Research Centre for Geosciences].

The meeting agenda featured 15-minute presentations over three days, describing new findings from G-FO observations and the combined GRACE and GRACE-FO [G/G-FO] climate data record that now spans over 21 years (2002–2023).
The meeting began with the customary G-FO project status session, covering programmatic mission and flight segment technical updates, future mission plans, and descriptions of the latest data released from the GRACE Science Data System (SDS) centers. Subsequent sessions featured more than 53 contributed presentations covering analyses, algorithms, and science results by Science Team members and attendees, totaling 57 oral and 5 poster presentations. Many of the presentations are posted on the GRACE website. While this summary will cover all the content on the agenda of the meeting – it does do so in an exact linear fashion. It begins with a G-FO mission status update, followed by key highlights from the contributed analysis and science presentations.
Status of GRACE Follow-On
Since their launch on May 22, 2018, the twin G-FO satellites have been tracking Earth’s water movements and global surface mass changes that arise from climatic, anthropogenic, and tectonic changes. G-FO also enables new insights into variations of ice sheet and glacier mass, land water storage, as well as changes in sea level and ocean currents. These measurements have important applications and implications for everyday life. The impact of these data is underscored by the publication of over 6000 scientific papers – an average of 5 new publications per week – that have established G/G-FO as a leading Earth Science mission.
In May 2023, G-FO successfully completed its Prime Mission phase that lasted five years after launch. G-FO was among the missions that went through the 2023 NASA Earth Science Senior Review . The NASA project team submitted its response in spring of 2023 to extend mission operations through 2026. The proposal received overall Excellent score, highlighting the unique utility the data provide for Earth Science research and societal applications. However, the G-FO project’s NASA budget will be reduced (compared to the previous baseline) by 15% in fiscal year (FY) 2024 and 24% in FY 2025 and 2026 due to the overall budget constraints that NASA is facing. The G-FO team remains confident in its ability to continue delivering high-value and high-impact science data products – prioritizing science operations management and data latency over data reprocessing campaigns. Both NASA and GFZ had already formally committed to extending their collaboration on G-FO mission operations and data processing through the end of 2026 via a Memorandum of Understanding.
As of December 2023, the G-FO project team has processed and released 62 monthly gravity fields – the most recent being for October 2023 (at the time of this writing). The primary mission objective for G-FO is to provide continuity for the monthly GRACE mass-change observations (2002–2017) via its Microwave Interferometer (MWI) intersatellite range-change observations. G-FO also demonstrated a novel technology demonstration Laser-Ranging Interferometer (LRI) for more accurate satellite-to-satellite ranging observations for future GRACE-like missions. The LRI has been successfully operated in parallel with the MWI for most of the mission, delivering excellent quality data. LRI-based monthly gravity and mass change fields covering the period from mid-2018 to mid-2023 have been made available by the SDS teams for further analysis and study by the science community.
Programmatic, Mission, and Operations Updates
The meeting began with Frank Flechtner [GFZ– German G-FO Project Manager ] and Felix Landerer [NASA/Jet Propulsion Laboratory (JPL)— U.S. G-FO Project Scientist ] giving welcoming remarks, followed by detailed assessments of the G-FO mission and operations status from the core SDS centers and flight operations teams.
GRACE Follow-On Project Status
Felix Landerer gave an overview of the G-FO satellites and the science data system performance. He reported that G-FO continues to meet its goal of extending the GRACE mass-change and gravity data record at equivalent precision and spatiotemporal sampling.
Since the previous STM in October 2022, the overall G-FO science instrument performance has been stable, and the SDS team continued to deliver a gapless monthly data record to users ahead of schedule (on average, within 43 days instead of the 60-day requirement). Improving the data calibrations of the accelerometer measurements – which are noise contaminated on one of the two G-FO spacecraft – remains a core focus of the project SDS team. To this end, an improved calibration approach that reduced data errors by 10–20% has been developed and will be operationalized by the team in the coming months.
Landerer reported that, as forecasted, the current Solar Cycle 25 has gained in strength through 2023 and will continue to do so through 2024 before subsiding again. The resulting higher non-gravitational forces acting on the satellites need to be properly accounted for in the accelerometer data processing.
He also noted that small thruster leaks in the satellites cold gas propulsion system have been closely monitored since 2021. To ensure stable data collection and sufficient lifetime margin to achieve continuity with the proposed successor mission GRACE-Continuity, or GRACE-C (which is the new name for the Earth System Observatory Mass Change mission scheduled for launch no earlier than 2028), the G-FO project team, in conjunction with guidance from the satellite manufacturer Airbus and the German Space Operations Center, decided to adjust the operational data collection mode of G-FO to a wide pointing mode – which means that the two spacecraft are allowed to deviate from their relative line-of-sight pointing by up to 2°, whereas the previous pointing angles were 100 times smaller. This operational change necessitates fewer thruster firings, which in turn reduces leaks and improves accelerometer calibrations – and thus leads to better overall science data quality. Due to the wide pointing, the LRI intersatellite ranging data collection has been suspended in this operational mode. However, the LRI instruments are still activated and fully functional. Landerer emphasized that reducing the leak ensures that the GRACE-FO mission will have sufficient fuel to remain operational up until GRACE-C launches.
Despite these operational challenges, Landerer said that the science data delivered by G-FO continues to provide excellent utility and insights into a rapidly changing Earth system. He briefly highlighted a few scientific and decision-support contributions and achievements of G-FO over the last year. These included:
- Monitoring California Groundwater . G-FO recorded the largest seasonal total water storage gains over California after the multiple atmospheric rivers made landfall during the 2022/2023 winter. Yet, peak water storage in May was below values observed 15–20 years ago – due to long-term, sustained groundwater declines. Going forward, the data will be invaluable to assess groundwater recharge rates and processes.
- Observing Water Cycle Extremes: Droughts and Pluvials . The G-GFO 20-year data record has been analyzed to show the increasing intensity of wet and dry extremes of the global water cycle, which increased as global temperatures rose.
- Tracking Polar Ice Mass Loss . G/G-FO measured net ice mass gains over Antarctica that began around 2021 due to snow accumulation mainly in East Antarctica, which offset the unabated mass loss of the West Antarctic ice sheet.
Subsequent science presentations presented in-depth analyses of these and other findings in the dedicated science sessions, some of which are summarized below.
Landerer also highlighted the expanding portfolio of open science contributions that the project team is supporting: Jupyter notebooks are part of an expanding GRACE Open Science toolbox with the goal to expand this toolbox with input from the Science Team and user community in the coming years. In addition, easy-to-use browser data portals at JPL and GFZ have been key to expand the science and applications user community that increasingly use the Level-3 and higher data products in decision support contexts (e.g., for drought monitoring and water resources management).
A series of status reports on programmatic G-FO mission operations, science operations, and SDS processing followed the opening presentations. Krzysztof Snopek [GFZ] reported on the ground and mission operations at the German Space Operations Center (GSOC), which is responsible for G-FO spacecraft operations. All essential flight operations, software updates, and planned calibrations were successfully scheduled and carried out by GSOC. Himanshu Save [University of Texas, Center for Space Research (CSR)] provided the science operations assessment. He described the evolving Solar Cycle 25 and its influence on the G-FO spacecraft, the mission’s fuel budget, and adjusted operational procedures and modes (such as the already-mentioned ‘wide’ pointing mode). Christopher McCullough [JPL] reviewed the status of G/G-FO Level 1 processing at JPL, detailing additional improvements made in the accelerometer calibrations. The team is using the noisy accelerometer data on one satellite and retrieving improved science information from it.
A representative from each of the G-FO mission SDS centers – which includes JPL, GFZ, CSR, and GSFC – summarized the status of the latest gravity-field and mass change data products [RL06.X L2], including an overview of background dealiasing models and the GFZ GravIS portal, the updated JPL mascon data product, new data-processing strategies, e.g., via range acceleration [CSR], and the status of ancillary Satellite-Laser-Ranging (SLR) data processing and dedicated G/G-FO products [GSFC].
Following the project team’s status presentations, there was a 30-minute session to answer questions from the science community and discuss in more detail the mission performance, near-term operations and data processing plans, as well as to gather suggestions and feedback from the community.
Science Presentations
The remainder of the sessions in the meeting were open-submission science sessions, each of which centered around different thematic topics, including: G/G-FO analysis techniques and next generation gravity mission (NGGM) concept studies, and science analysis of mass-transport data in the fields of glaciology, oceanography, hydrology, and solid-Earth physics. As has been the case in previous years, the presenters underscored the value of interdisciplinary and multi-instrument analyses that utilize the unique complementary value of G/G-FO mass-change observations in combination with other remote sensing data (e.g., satellite altimetry or precipitation observations) and in situ data (e.g., surface deformation or ocean temperature profiles). Such hydrogeodetic combinations yield improved spatial and temporal resolutions that enable advances in Earth system process understanding, which increasingly advance societal applications of science results in support of NASA’s programmatic focus on Earth Science to Action , which seeks to “advance and integrate Earth science knowledge to empower humanity to create a more resilient world.”
Section A: GRACE and GRACE-FO Geodesy
The project status reports presented under the previous heading were part of the first section of the agenda (Session A1) as were two additional sessions: Analysis Techniques and Intercomparisons (Session A2) and NGGM and Bridging the Gap (Session A3), which focused on plans, concepts, and technologies being developed for future gravity missions. Highlights from each of these two sessions follow in the next two subsections.
Analysis Techniques and Inter-comparisons
This session featured 15 presentations by the SDS centers and ST members on progress in instrument data calibrations and novel data processing algorithms and methods, including data-fusion with other observations.
Representatives from G/G-FO processing centers presented updated gravity-field time-series data, which capitalize on improved parameterizations, better instrument error characterizations (e.g., from star cameras, accelerometers, or ranging instruments) and background models (e.g., for tides) for improved monthly mass change data and uncertainty quantification. The highly accurate LRI data provides further opportunities to identify and characterize measurement system errors, which can be exploited for G-FO data processing but is also informative in the development of the future GRACE-C mission. However, it was also shown that several metrics used in identifying gravitational errors are sensitive to the estimated satellite trajectory, and consequently a sufficient understanding of the orbital trajectory is necessary to make accurate adjustments to the gravity field based on satellite observations.
The G/G-FO data products make use of ground-based geodetic observations, such as satellite laser-ranging (SLR) to a network of dedicated SLR satellites, which can be used to extend the G/G-FO interannual data record back to ~1994 – albeit at a much-reduced spatial resolution. Additionally, SLR data provide an important validation and performance assessment opportunity for G/G-FO observations. In that regard one presenter showed results indicating the recent G-FO accelerometer updates have indeed resulted in better gravity and mass change fields. Other speakers discussed the value and potential for improvement that could be achieved by combining G-FO and SLR observations more formally to exploit the data strengths of the different observation types in an optimal way. Such approaches could reduce uncertainties in global ocean and land ice mass changes. Furthermore, deployment of stable, long-term ocean bottom pressure (OBP) recorders in the Arctic Ocean in 2022 has enabled progress on G/G-FO OBP data validation. The data from these OBP recorders are entirely independent of G/G-FO observations and are thus very valuable to assess the satellite data record. An initial comparison between 1.5 years of OBP data and various G-FO OBP products suggest excellent agreement.
The data collected from G/G-FO has a native resolution of about 300 km (~186 mi). By jointly analyzing these G/G-FO data with higher-resolution surface elevation changes from a multimission synthesis of radar and laser satellite altimeters, net mass changes can be effectively downscaled (within a Bayesian framework ) to less than 20 km (~12 mi) resolution, which is sufficiently high resolution to resolve individual ice streams in Antarctica that cannot be separated using G/G-FO data alone.
NGGM and Bridging the Gap
The presenters in this session provided status-update on the GRACE-C mission, a joint project between NASA and the Deutsches Zentrum für Luft- und Raumfahrt (DLR) [German Aerospace Center], as well as on future instrument developments and mission concepts.
The 2017 NASA Earth Science Decadal Survey Report highlighted mass-transport monitoring through gravity change as one of five designated observables (i.e., top priorities for study) in Earth observations for the next decade in collaboration with international partners. The GRACE-C project successfully passed the NASA/JPL Mission Concept Review in June 2022, and the NASA Key Decision Point B review in September 2023 and is currently in its Phase B project definition phase. GRACE-C will be a single satellite pair based on a fully redundant LRI (as demonstrated on GRACE-FO) in a polar orbit at 500 km (~311 mi) altitude. To avoid a data gap after GFO, a launch date of no later than 2028 is targeted for GRACE-C.
Similarly, GFZ has been conducting model simulation studies to determine the value of adding a second satellite pair, dubbed Next-Generation Gravity Mission (NGGM) in Europe. The experiments reveal that advanced parameterization techniques for improved de-aliasing of short-term mass variations can significantly reduce data errors and open the possibility for higher spatial and temporal resolution data products and science applications.
The technology demonstration LRI on G-FO has surpassed its performance requirements. With a LRI expected to be the primary instrument for the GRACE-C mission as well as other future GRACE-like missions, development of a new technique is required to provide long-term laser frequency knowledge to provide a scale correction factor to the geodesy measurement. The LRI-team presented updated results of a so-called scale factor measurement technique that allows the accurate determination of the laser frequency on-orbit that can meet the stringent GRACE-C mission requirements. This was achieved with a dual frequency modulation scheme, and a prototype electronics unit has been developed and tested, demonstrating performance better than the expected mission requirements.
There were also reports on progress in technology development of low-frequency optomechanical accelerometers for geodetic applications. These highly-sensitive, compact, portable – and cost-effective – optomechanical inertial sensors build upon recent advances in optomechanics to measure accelerations with small form factors. The development of a sensor with lower cost, size, weight, and power – yet with GRACE-like performance – is a major achievement as these could be integrated into cost-effective mission designs, spacecraft miniaturization, simplified architectures, as well as for the deployment of constellations of satellite pairs flying at lower altitudes.
Section B: Geophysics and Climate Science
There were five sessions included in this section of the agenda, which are summarized in the subsections below as follows: Hydrology (Session B4), Cryosphere (Session B2), Solid Earth Sciences (Session B1), Oceanography (Session B3), and Multidisciplinary Science (Session B5).
Hydrology
This session, with 12 presentations, highlighted advances in hydrology research and applications using G/G-FO data enabled by the unique value of long, uninterrupted mass change climate data record.
The topic of terrestrial water storage variations in California came up in several presentations, focusing on the see-saw swings between very wet and very dry years and the early impacts on groundwater recharge after the record-breaking snow accumulation during the 2022/2023 winter. The process of groundwater recharge – an important objective in the 2017 Earth Science Decadal Survey – is not well understood because of the challenges in observing infiltration of new water supply into the ground and the effects of rate of input, amount of input, and various aquifer characteristics. By combining observations of precipitation, snow water equivalent, surface water storage, ground surface deformation, and groundwater storage from G/G-FO, recharge behavior can be characterized in a natural experiment where source inputs are effectively not limited, but recharge capacity is limited. Results of studies shown during the meeting reveal that only a fraction of total available potential recharge can enter the aquifer, and that G/G-FO observations allow us to measure the effective aggregated recharge capacity and how it varies with several predictors. Another paper reported that subsurface water increases in California’s Sierra Nevada by 0.6 m (~2 ft) from October 2022 to June 2023, which represents 43% of the cumulative precipitation.
Several presenters reported on efforts to advance concepts to downscale G/G-FO data to bring the information closer to decision-making scales and expand water-related applications, as well as to fill gaps and expand the data record with multisensor observations. One presenter described a new spectral approach that employs wavelet multiresolution analysis to combine seasonal terrestrial water storage change data from G/G-FO with those from global navigation satellite system (GNSS) ground station networks to downscale the observations to smaller hydrological basins and to better separate processes over complex topographical terrain. This method can also be used by fusing G/G-FO and hydrological model data [e.g., from NASA’s Global Land Data Assimilation System (GLDAS) models at continental scales]. Importantly, the method yields trends and long-term signals that match G/G-FO observations – a strength of the observing system. Another approach used a statistical Bayesian framework to incorporate G/G-FO observations and Soil Moisture Change data from different available sources [e.g., NASA’s Soil Moisture Active Passive (SMAP) mission] to obtain nonparametric likelihood functions that allow for downscaling. A statistical technique called cyclostationary empirical orthogonal function (CSEOF) analysis – which is used to interpret space-time variability in a large dataset – allowed researchers to fill short data gaps (~1 year) in G/G-FO record (e.g., between 2017 and 2018 – the gap between GRACE and GRACE–FO) without having any additional data. With the support of physically-related data (e.g., precipitation and temperature), CSEOFs can be used to reconstruct water changes into the past or fill larger data gaps. Such datasets improve understanding of trends and natural variability and anticipate future trends in response to climatic changes.
Another presenter described a science study that found an apparent abrupt decline in temperate (non-ice) Terrestrial Water Storage (TWS) in 2015 to a new, lower regime that appears to be unique in the past 33 years. The triggering event for this new lower TWS regime appears to be the massive drought in Brazil in 2015. Subsequent droughts around the world (e.g., Europe, the western U.S., Canada, central Africa, and southern Brazil) have helped to keep TWS values depressed. Warm global sea surface temperatures, prevalent since 2015, have decreased rain accumulation over the continents, reducing TWS.
In the European Alps region, a G/G-FO data analysis found that glacier and ice changes are the major contributors to the observed signals. Overall, glaciers here have lost ice mass at rates between 1.4 to 2.2 Gt/year since 2002. Advances in spatial downscaling and data combinations are expected to allow for improved estimates and applications, including geological hazard monitoring.
In Northern Italy, accelerated groundwater loss has been detected using G/G-FO, well measurements, and vertical land motion observations. Since 2015, the groundwater loss has accelerated. Assuming a best-case scenario (conditions similar to 2007–2014), it could take 13–28 years for ground water storage to recover from recent long-term period of decline, thus setting the stage for prolonged drought conditions.
Since a pioneering study in 2014, it is well-established that G/G-FO observations of TWS are an effective means to estimate flood potential and flood risks due to water-saturated soil. Novel G/G-FO data processing schemes that exploit sub-monthly variations of total water storage enabled researchers to delineate basin-specific storage-discharge dynamics more accurately. They found that at submonthly timescales in many global basins, water storage (i.e., saturated soil) has more impact on whether a flood will occur than the amount of precipitation that falls.
Along the Nile River, G/G-FO data were used to monitor water changes in crucial artificial reservoirs. These data indicate that water losses through underground-seepage over the geologically highly fractured region via a complex network of shear systems, faults, and fractures, are significant and could impact the delicate water balance in the region. A separate study focusing on nearby Southern Arabia found that intense tropical cyclones (wind speeds > 64 kph or ~40 mph) have doubled in the past decade compared to the preceding two, which resulted in significant recharge of the aquifers in the study area. The findings demonstrate the ability of G/G-FO to capture recharge signals and monitor aquifer systems in poorly gauged basins and highlight the significant role of tropical cyclones in recharging aquifers in arid Arabia.
The five contributions in this session reported on new ice mass balance results of the Earth’s land-ice, as well as on novel data-combinations approaches that can improve the spatial resolution over G/G-FO-only data.
The Antarctica Ice Sheet contributes to the largest sea level rise potential and remains as the largest uncertainty source in the prediction of future sea levels. Data from G-FO and the Ice, Clouds and land Elevation Satellite–2 (ICESat-2) mission have been used to track ice sheet mass and height changes in Greenland and Antarctica, respectively. By combining the strengths of G-FO (gravity or mass change) and ICESat-2, (laser altimetry) data, a more accurate and less uncertain estimate of ice sheet mass changes can be achieved. This combination has led to a proposal for an enhanced iterative algorithm for deriving Antarctic mass balance, incorporating key technologies such as altimetry, gravity measurements, Global Positioning System (GPS) satellite data, and surface mass balance models. The study utilizes an effective density map derived from ICESat-2 and tests the algorithm’s sensitivity and uncertainty with synthetic data, considering realistic physical processes and variability. This approach aims to address discrepancies in estimating ice mass loss in East Antarctica and provides important guidance for optimizing future ground measurements (i.e., GPS station positions). Another presentation focused on understanding the differences in mass change recovered by the G/G-FO and IceSat-2 missions – both in terms of spatial distributions and total magnitudes – to ultimately determine a best combined estimate of ice sheet mass change leveraging the strengths of each mission.
Temporal gravity field estimates from G/G-FO data reveal that the Antarctic ice sheet contributed approximately 6.1 mm (~0.2 in) to global sea level rise from 2002–2022, with a net loss of ~2150 GT of mass. While mass change accelerated during the GRACE era, it has decelerated during the GRACE-FO era – due to increased mass gain in East Antarctica. The deceleration is attributed to surface mass balance processes: annual precipitation and increased incidences of extreme weather events in East Antarctica, challenging predictions based on correlations with climate indices like Southern Annular Mode and El Niño Southern Oscillation.
A related study confirmed a pause in Antarctica’s mass loss, a non-accelerating mass loss in Greenland, and a steady loss from glaciers and ice caps away from the poles. The use of the LRI observations enabled novel submonthly analysis in key regions (including the Amundsen Sea Embayment of West Antarctica and the Pine Island/Thwaites basins) to gain more understanding of fast ice dynamics and their spatial extent.
While G/G-FO data span two decades, estimates of Earth’s oblateness from other satellite observations that date back to 1976 and provide a much longer data record – albeit at much coarser spatial resolution. This half-century long timeseries provides important constraints on ice mass change prior to the launch of GRACE in 2002. The data suggest that ice mass loss had already begun to accelerate by the 1990s. Recent progress in Earth system models, in conjunction with the long satellite data record, are being used to isolate trends in glacial isostatic adjustment (GIA) – which is the vertical movement of the Earth’s surface after the weight of glaciers is removed from them – and to improved estimates of ice mass loss prior to GRACE.
Solid Earth Sciences
Two presenters in this session described their efforts to evaluate signals in the G/G-FO data record associated with earthquakes. The G/G-FO data provide a unique opportunity to observe the Earth’s response to great earthquakes across diverse tectonic settings at time scales from days to decades. Using 13 earthquakes of magnitude (Mw)>8.0 over the last 20 years, it was found that elastic bulk modulus and viscosity govern large-scale coseismic and postseismic gravimetric changes, respectively. By constraining the solid Earth’s viscosity structure, improved physics-based models of long-term postseismic changes can be developed that incorporate observations from G/G-FO. The portion of the long-term gravity change signal that can be attributed to these earthquakes can then be removed from the G/G-FO data to better quantify processes related to ocean mass and hydrology changes. When physics-based models are not available, alternative statistic-based approaches can be used to remove the co- and post-seismic signature of large earthquakes (e.g., 2004 Andaman-Sumatra and 2011 Tohoku, Japan quakes) from the G/G-FO data.
As the G/G-FO data record extends into its third decade, the long time series of Earth gravity changes requires careful consideration of the solid-Earth response to contemporary surface mass changes. To isolate the gravity signature of any surface mass signal, it is becoming evident that simple elastic loading corrections are no longer sufficient. Recent advances in mantle rheology – describing and understanding the nature of Earth’s mantle – derived in mineral laboratory experiments, tidal modeling, and seismic imaging provide unequivocal evidence of anelastic contributions to solid-Earth deformation on time-scales ranging from hours to decades. New developments in the solid-Earth capabilities of JPL’s Ice-sheet and Sea-level System Model (ISSM) in the form of viscoelastic solvers for Love numbers and sea-level change was used to implement and explore the so-called Extended Burgers Material (EBM) and so resulting viscoelastic deformations between the seismic and GIA time scales. Preliminary testing with EBM rheology shows potential for a ~15–20% increase in mass change trends for some regions.
A subdecadal variation of large-scale (i.e., spanning over continental scales) gravity signals with a period of approximately six years has attracted intense interest in the geodesy and geodynamic communities. Earth’s fluid core motions, magnetic field, Earth rotation, and crustal deformations have been invoked as causes for this signal. An analysis of G/G-FO data showed that a significant part of the approximately six-year signals is in fact due to climate-related oscillation of ocean-atmosphere coupling in the Pacific and Atlantic and variations in the land water storage over Africa.
Oceanography
In the oceanography session, five presenters reported on the combination of G/G-FO, satellite altimeters (e.g., from the joint NASA–European Sentinel-6 Michael Freilich mission), and in situ ocean floats (e.g., Argo ) to investigate variations in sea level and ocean circulations – e.g., see Figure 1 . Other presenters discussed improvements in data processing by reducing errors in atmospheric tides that could lead to spurious trends or double-counting a subset of ocean tides and by incorporating new dedicated ocean data grids that remove geodetic signals not related to ocean dynamics (e.g., global ocean mass; large earthquake signals).
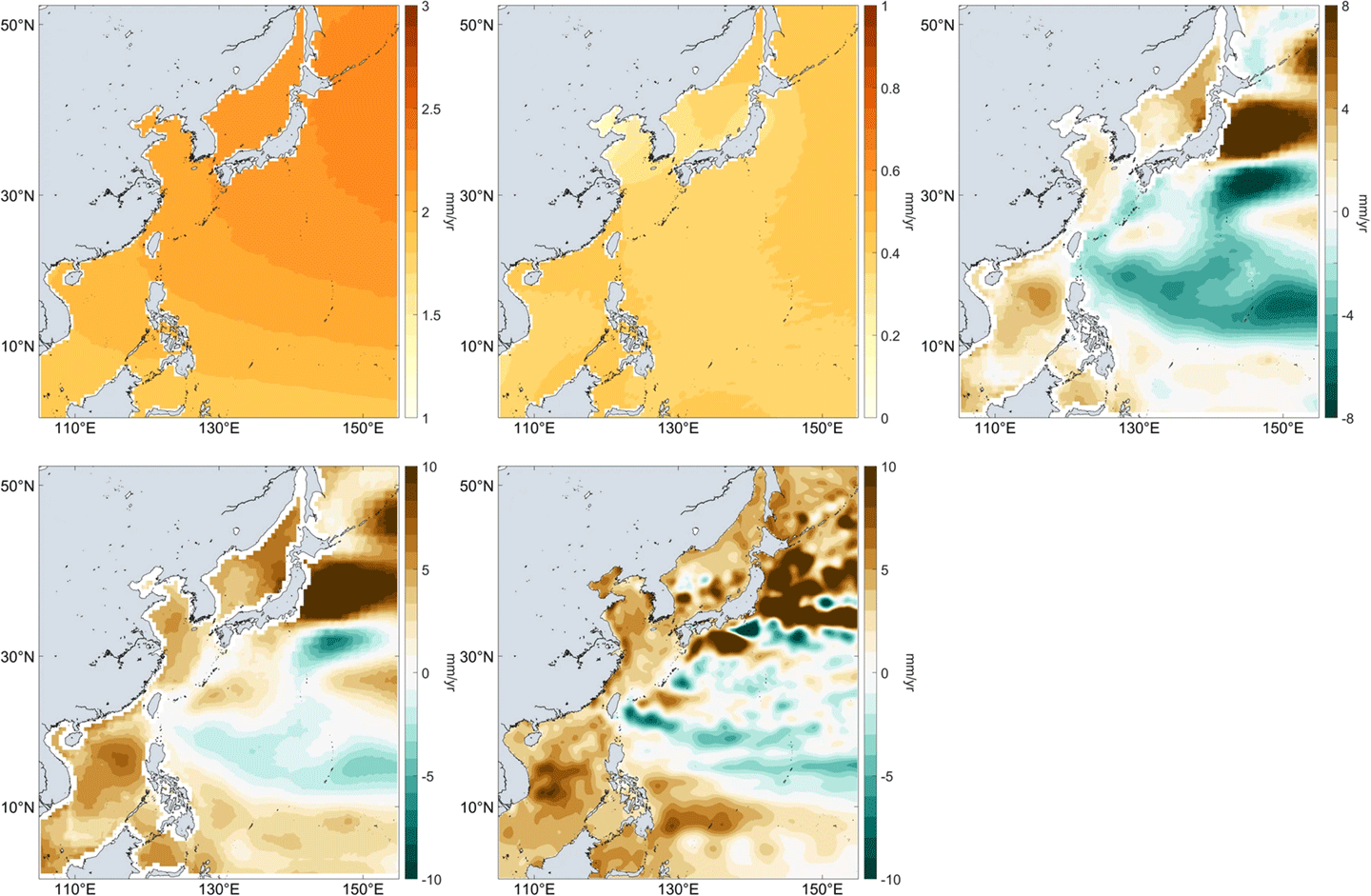
Another presenter described how ocean mass redistribution and regional sea-level rise in the North-West Pacific marginal seas (i.e., around Japan and north of the Philippines) is impacted by seafloor deformation from earthquakes, which alter the ocean bathymetry. G/G-FO data are key to isolating these deformation effects, which in turn allows better sea level projections that can be used for planning purposes.
While long-term sea level trends are of major concern, the seasonal cycle is the dominating climate signal in ocean bottom pressure variability. Accurate representation of seasonal cycle is thus key to efforts to improve observations and models of ocean bottom pressure. Examining differences between models and observations elucidates remaining uncertainty in observations and missing physics in the models (e.g., lack of intrinsic variability due to coarse resolution, no accounting of gravitational and loading effects). This allows researchers to advance the quality of ocean mass change observations and unravel underlying dynamics.
Lastly, ocean bottom pressure observations from G/G-FO have been used to monitor transport variability of deep currents associated overturning circulation in the Northern Hemisphere (the Labrador Current) and Southern Hemisphere (Weddell Sea Bottom Water). This deepwater transport provides an important pathway for the sequestration of excess atmospheric heat and carbon from locations of water mass formation. Continuous observations of deep ocean currents provide valuable insight into Earth’s climate system. However, harsh conditions and complex recirculation transport pathways make in-situ observations of these deep flowing currents challenging.
Interdisciplinary Science
Six presenters contributed to this session. The first study revisited geodetic assumptions about measuring so-called Earth Center-of-Mass (CM) motions that can be traced to planetary-scale seasonal and long-term variations of water cycling between the land the oceans. Differences in SLR and G/G-FO estimates of CM estimates can be helpful to refining global circulation models. In a related study, G/G-FO and SLR data have been used to pin down the causes and origin of polar motion, particularly the mass component related to gravity changes. A novel hybrid SLR/GRACE time-variable gravity approach closely aligned well with the hydrological excitation in independently polar motion.
Errors in GIA corrections impact altimeter estimates of sea level and ocean mass estimates and the so-called sea level budget . Choices in modeling GIA, particularly based on paleoshoreline sites, affect Earth’s viscosity structure and GIA response, influencing global mean sea level (GMSL) budget closure. Even minor Earth model changes can have notable effects on the alignment of GMSL (altimetry), ocean mass (GRACE), and steric sea level change (Argo). Thus, future research needs to focus on accounting for the complex three-dimensional structure of the solid Earth to improve GIA corrections and more accurately isolate contemporary mass change in the G/G-FO data record.
Despite GIA uncertainties, G/G-FO, in combination with sea level measurements from altimetry, provide a unique capability to measure changes in ocean heat content. The ocean takes up nearly 90% of Earth’s current energy imbalance, signifying their important role in overall planetary heating. Two presenters reported consistent findings of ocean heat uptake rates of 0.9 W/m 2 based on the indirect geodetic satellite measurements of sea level and ocean mass – a value that is entirely independent of other techniques and thus provides crucial validation – see Figure 2 . In addition, the results indicated the overall heating rate over the last decade has increased, which means heat accumulation is accelerating.
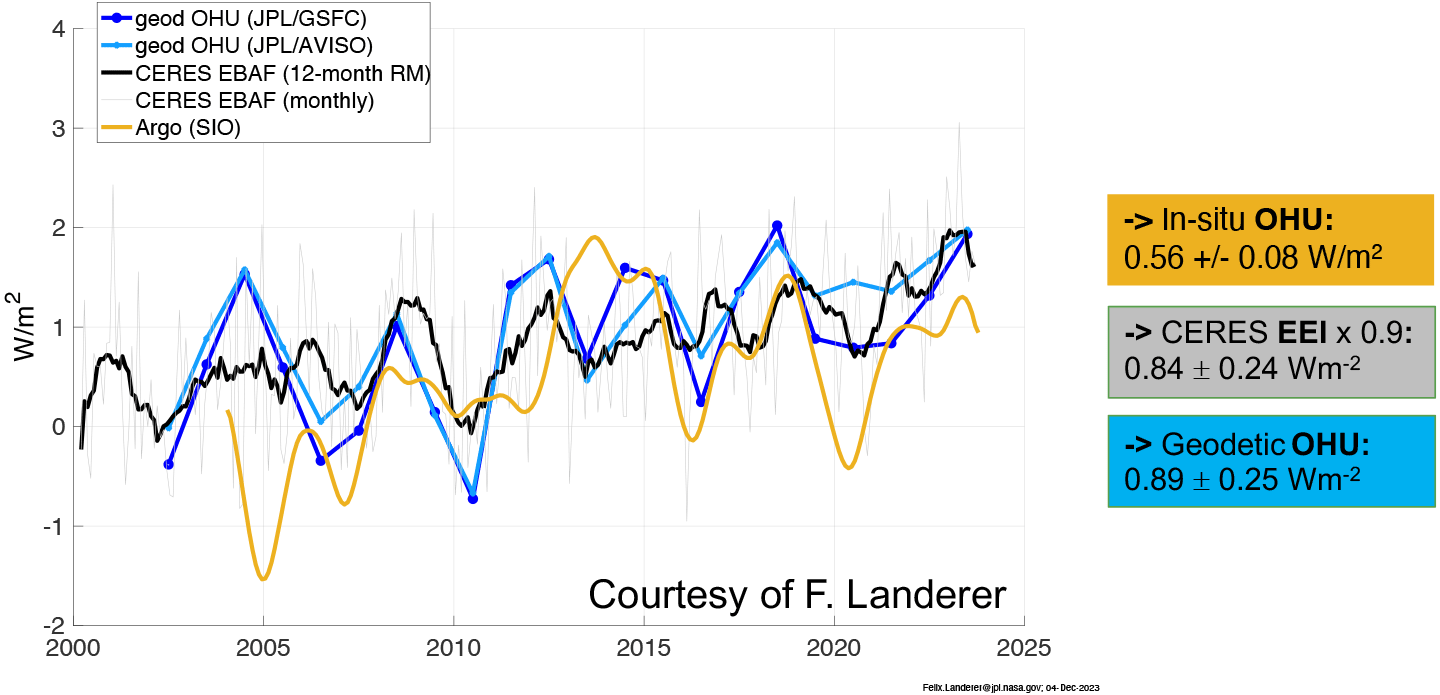
The hybrid 2023 G-FO STM brought together over 120 international participants and showcased a broad range of science results and applications that are supported and uniquely enabled by the satellite gravimetry-based mass change observations. The G-FO data now span nearly six years and continue to provide crucial insights into how Earth’s hydrosphere, including sea level, ocean currents, and water distribution over land, is changing. The G/G-FO data are extending important climate data records (e.g., the Greenland and Antarctic ice mass time-series, ocean mass sea level data, and TWS over land) into their third decade. The upcoming GRACE-C mission will build on and expand this mature data record, which is increasingly enabling important applications in support of water-related decision making and planning.
The G-FO project team remains focused on providing the mass-change data record at a level of performance consistent with that of GRACE. As the current Solar Cycle 25 increases towards its anticipated maximum in 2024, the team continues to improve the mission’s accelerometer data products in support of that goal. Corresponding data improvements in the monthly gravity and mass change products will be released early 2024.
The next G-FO STM will be held from October 8–10, 2024 in Potsdam, Germany, organized by GFZ. Check the GRACE website for specific details as the date gets closer.
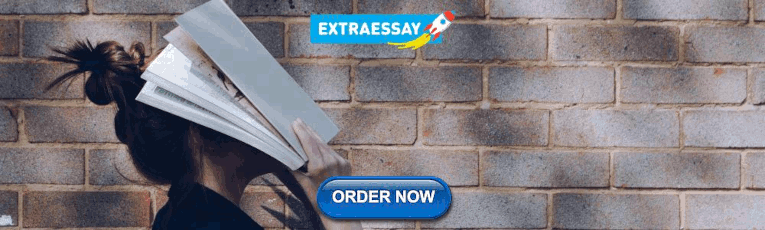
IMAGES
VIDEO
COMMENTS
A graphical distribution of the locations of water on Earth Visualisation of the distribution (by volume) of water on Earth. Each tiny cube (such as the one representing biological water) corresponds to approximately 1,000 km 3 of water, with a mass of about 1 trillion tonnes (200,000 times that of the Great Pyramid of Giza or five times that ...
Notice how of the world's total water supply of about 332.5 million cubic miles of water, over 96 percent is saline. And, of the total freshwater, over 68 percent is locked up in ice and glaciers. Another 30 percent of freshwater is in the ground. Fresh surface-water sources, such as rivers and lakes, only constitute about 22,300 cubic miles ...
Diagram of Water Distribution on Earth. Of the 70 percent of the planet water covers, 97.5 percent is saltwater. Although water covers about 70 percent of Earth's surface, only a small percentage of that water is freshwater, and even less of that is easily accessible to the billions of organisms that depend on freshwater for survival.
The World's Water - Distribution of Earth's Water. The Earth is a watery place. But just how much water exists on, in, and above our planet? About 71 percent of the Earth's surface is water-covered, and the oceans hold about 96.5 percent of all Earth's water. Water also exists in the air as water vapor, in rivers and lakes, in icecaps and glaciers, in the ground as soil moisture and in ...
Exercise 5.1.1 5.1. 1. The hydrosphere contains about 1.36 billion cubic kilometers of water, and 71% of the earth's surface is covered with water. Conduct some research and fill in the appropriate distribution of the earth's hydrosphere: The water cycle is an open system.
The Water Cycle. Earth's oceans contain 97% of the planet's water, so just 3% is fresh water, water with low concentrations of salts. Most fresh water is trapped as ice in the vast glaciers and ice sheets of Greenland. A storage location for water such as an ocean, glacier, pond, or even the atmosphere is known as a reservoir.
Distribution of the Earth's Water. The distribution of water on the Earth's surface is extremely uneven. Only 3% of water on the surface is fresh; the remaining 97% resides in the ocean. Of freshwater, 69% resides in glaciers, 30% underground, and less than 1% is located in lakes, rivers, and swamps. Looked at another way, only one percent of ...
6.1: Distribution of Earth's Water. Water is composed of two atoms of hydrogen and one atom of oxygen bonded together. Despite its simplicity, water has remarkable properties. Water expands when it freezes and has high surface tension because of the molecules' polar nature that they tend to stick together.
Water Distribution. The total volume of water on Earth is estimated at 1.386 billion km 3 (333 million mi 3), with 97.5% being salt water and 2.5% being fresh water.Of the fresh water, only 0.3% is in liquid form on the surface (USGS 2016; Eakins and Sharman 2010; Gleick 1993).. In addition, the recent theories and research assume the high water storage capacity of minerals in Earth's mantle ...
The water cycle describes where water is on Earth and how it moves. Water is stored in the atmosphere, on the land surface, and below the ground. It can be a liquid, a solid, or a gas. Liquid water can be fresh or saline (salty). Water moves between the places it is stored. Water moves at large scales, through watersheds, the atmosphere, and ...
As we've already covered in Module #1, this water is distributed in the oceans, ice caps and glaciers, surface water (streams, lakes, and rivers), groundwater, soil moisture, the atmosphere, and in biomass. However, these reservoirs of Earth's water are not static; water is constantly fluxing between them. We see this transport of water ...
water distribution on Earth. • Students will understand that most of the water on Earth is not readily available for human use or consumption because of salinity, location or pollution. • Students will understand that most of Earth's fresh water is unavailable, locked in glaciers and ice caps. • Students will understand that most of the
The Water Cycle. By Steve Graham, Claire Parkinson, and Mous Chahine Design by Robert Simmon October 1, 2010. Viewed from space, one of the most striking features of our home planet is the water, in both liquid and frozen forms, that covers approximately 75% of the Earth's surface. Geologic evidence suggests that large amounts of water have ...
As the number of people rises, our need for water will grow. By the year 2025, only half the world's people will have enough clean water. Water is such a vital resource that serious water shortages may cause other problems. Crops and livestock may die, so people will have less food available. Other uses of water, such as industry, may have to ...
Distribution of Earth's Water. Earth's oceans contain 97% of the planet's water, so just 3% is fresh water, water with low concentrations of salts. Most fresh water is trapped as ice in the vast glaciers and ice sheets of Greenland. A storage location for water such as an ocean, glacier, pond, or even the atmosphere is known as a reservoir.
Explore LearningMedia Resources by Subject. Learn about the distribution of water on Earth with these NASA and USGS graphics. This resource enables students to visualize and interpret data on water availability and distribution.
Earth's water distribution. Image. Add to collection. Tweet. Rights: The University of Waikato Te Whare Wānanga o Waikato Published 15 April 2009 Size: 110 KB Referencing Hub media. Over 70% of the Earth's surface is covered in water, yet most of this is made up of seawater in oceans. Just a small amount is freshwater.
Classroom Lesson: Water World. In this lesson, students explore the water cycle and where humans live relative to water, learn about Earth's water distribution, get to know a river or stream in their neighborhood, and think about river travel today and in the past. This lesson is intended for grades 4-6 and is aligned to NGSS.
About 71% of the earth's surface is covered with water. Of the total volume of water on Earth: 97% is saltwater 2% is freshwater frozen in ice caps and glaciers 1% is fresh water in lakes and streams, groundwater, and water vapor in the atmosphere In general, most of the earth's water is located in the oceans as saltwater. Most
Students describe and generate charts to answer a scientific question on differences in volume between fresh water and salt water. Students consider why a given graph, while accurate, does not provide the information needed to answer a specific question about the distribution fresh and salt water on Earth.
Notice how of the world's total water supply of about 332.5 million cubic miles of water, over 96 percent is saline. And, of the total freshwater, over 68 percent is locked up in ice and glaciers. Another 30 percent of freshwater is in the ground. Fresh surface-water sources, such as rivers and lakes, only constitute about 22,300 cubic miles ...
Learn about the distribution of water on Earth and how available and accessible freshwater is used and replenished by precipitation with this NASA video and graphic. This resource provides opportunities for students to ask questions as well as plot and analyze data on the distribution and uses of water on Earth.
The largest sphere represents all of Earth's water. Its diameter is about 860 miles (the distance from Salt Lake City, Utah, to Topeka, Kansas) and has a volume of about 332,500,000 cubic miles (mi 3) (1,386,000,000 cubic kilometers (km 3 )). This sphere includes all of the water in the oceans, ice caps, lakes, rivers, groundwater, atmospheric ...
Since their launch on May 22, 2018, the twin G-FO satellites have been tracking Earth's water movements and global surface mass changes that arise from climatic, anthropogenic, and tectonic changes. G-FO also enables new insights into variations of ice sheet and glacier mass, land water storage, as well as changes in sea level and ocean currents.
Ransomware is a type of malicious software that encrypts or locks user files and demands a high ransom. It has become a major threat to cyberspace security, especially as it continues to be developed and updated at exponential rates. Ransomware detection technology has become a focus of research on information security risk detection methods. However, current ransomware detection techniques ...