June 16, 2008
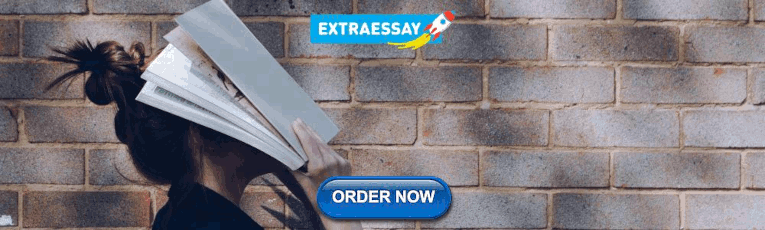
Were Meteorites the Origin of Life on Earth?
DNA building blocks found in meteorite took shape in deep space
By JR Minkel
On supporting science journalism
If you're enjoying this article, consider supporting our award-winning journalism by subscribing . By purchasing a subscription you are helping to ensure the future of impactful stories about the discoveries and ideas shaping our world today.
A new study finds that a pair of chemical building blocks similar to those in genetic material was present in a meteorite before it fell to Earth in the 1960s. Researchers say the finding makes it slightly more plausible that meteorite bombardments may have seeded ancient Earth with life's raw materials, potentially paving the way for life itself. Part of the scientific mystery of how life emerged is the origin of chemical building blocks: Were they created by chemical reactions on Earth or did they, perhaps, hitch rides on meteorites that may have germinated our and other planets in our solar system with the same molecules? By studying meteorite fragments, such as those that fell in 1969 near the town of Murchison, in the southeastern Australian state of Victoria, researchers have learned that they contain carbon-based compounds that most likely formed in space, including sugars and amino acids. They were not sure, however, about a class of compounds called nucleobases, which when fused with sugar molecules are the building blocks of nucleic acids such as DNA (the stuff of genes) and its close cousin RNA (produced when genes switch on). Researchers have speculated that life may have arisen from RNA molecules that acquired the ability to copy themselves. But they have had a hard time generating nucleobases in experiments designed to mimic chemical conditions on the early Earth, says Zita Martins, a chemist and astrobiologist at Imperial College London. To check the origin of two nucleobases—uracil, found in RNA, and xanthine, a common cellular constituent—Martins and her colleagues analyzed the ratio of carbon isotopes in the two compounds. Most carbon on Earth consists of carbon 12, named for the number of protons and neutrons in its atomic nucleus, rather than its slightly heavier cousin, carbon 13. Martins and her colleagues compared carbon isotopes in the Murchison nucleobases to soil samples from Murchison as well as to a common mineral. As expected if the nucleobases were forged in deep space, they were richer in carbon 13—by 44.5 percent for uracil and 37.7 percent for xanthine—compared with the other samples, the group reports in Earth and Planetary Science Letters . "It really clarifies at least that the building blocks of genetic material, the nucleobases, were available" in the early Earth, Martins says. "We are not saying that only meteorites contributed to the building blocks of life," she adds, "but it's a very great contribution." The concentration of nucleobases in the Murchison meteorite is relatively low. Martins and her co-workers needed 0.5 ounce (15 grams) of space rock to extract their sample, compared with milligram-size samples for other chemicals, she says. But researchers believe that space rocks and dust once rained onto Earth in billions of tons per year. Other researchers say the finding appears to be solid, although some are skeptical of its significance. Robert Shapiro, a professor emeritus and senior research scientist in chemistry at New York University, says that because of their low concentration, extraterrestrial nucleobases were unlikely to have played much of a role in kick-starting life. "They're a subunit of a subunit of DNA," he says. "My opinion is that their amounts were utterly unimportant and insignificant." He says he would be more impressed if whole nucleosides—bases plus sugars—were found in meteorites in concentrations similar to those of amino acids. And researchers may yet discover ways that Earthly chemistry—perhaps around hydrothermal vents —could have generated nucleobases and other compounds. Conel Alexander, a geochemist at the Carnegie Institution of Washington who specializes in meteorites, says that without more data, claims about the amounts and sources of molecules on early Earth should be taken with a grain of salt. "It really comes down to quantitative arguments about how much was made on Earth [and] how much was brought in from space," he says. "Any honest person would keep an open mind about the whole issue."
Correction (6/17/08): This article originally stated incorrectly that isotopes are named for the number of neutrons in their nuclei, instead of the total number of protons and neutrons.
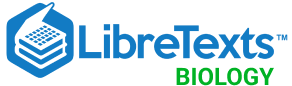
- school Campus Bookshelves
- menu_book Bookshelves
- perm_media Learning Objects
- login Login
- how_to_reg Request Instructor Account
- hub Instructor Commons
Margin Size
- Download Page (PDF)
- Download Full Book (PDF)
- Periodic Table
- Physics Constants
- Scientific Calculator
- Reference & Cite
- Tools expand_more
- Readability
selected template will load here
This action is not available.
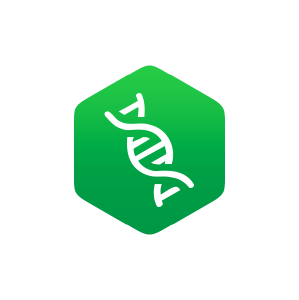
18.9: The Origin of Life
- Last updated
- Save as PDF
- Page ID 5932
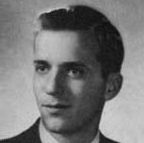
- John W. Kimball
- Tufts University & Harvard
\( \newcommand{\vecs}[1]{\overset { \scriptstyle \rightharpoonup} {\mathbf{#1}} } \)
\( \newcommand{\vecd}[1]{\overset{-\!-\!\rightharpoonup}{\vphantom{a}\smash {#1}}} \)
\( \newcommand{\id}{\mathrm{id}}\) \( \newcommand{\Span}{\mathrm{span}}\)
( \newcommand{\kernel}{\mathrm{null}\,}\) \( \newcommand{\range}{\mathrm{range}\,}\)
\( \newcommand{\RealPart}{\mathrm{Re}}\) \( \newcommand{\ImaginaryPart}{\mathrm{Im}}\)
\( \newcommand{\Argument}{\mathrm{Arg}}\) \( \newcommand{\norm}[1]{\| #1 \|}\)
\( \newcommand{\inner}[2]{\langle #1, #2 \rangle}\)
\( \newcommand{\Span}{\mathrm{span}}\)
\( \newcommand{\id}{\mathrm{id}}\)
\( \newcommand{\kernel}{\mathrm{null}\,}\)
\( \newcommand{\range}{\mathrm{range}\,}\)
\( \newcommand{\RealPart}{\mathrm{Re}}\)
\( \newcommand{\ImaginaryPart}{\mathrm{Im}}\)
\( \newcommand{\Argument}{\mathrm{Arg}}\)
\( \newcommand{\norm}[1]{\| #1 \|}\)
\( \newcommand{\Span}{\mathrm{span}}\) \( \newcommand{\AA}{\unicode[.8,0]{x212B}}\)
\( \newcommand{\vectorA}[1]{\vec{#1}} % arrow\)
\( \newcommand{\vectorAt}[1]{\vec{\text{#1}}} % arrow\)
\( \newcommand{\vectorB}[1]{\overset { \scriptstyle \rightharpoonup} {\mathbf{#1}} } \)
\( \newcommand{\vectorC}[1]{\textbf{#1}} \)
\( \newcommand{\vectorD}[1]{\overrightarrow{#1}} \)
\( \newcommand{\vectorDt}[1]{\overrightarrow{\text{#1}}} \)
\( \newcommand{\vectE}[1]{\overset{-\!-\!\rightharpoonup}{\vphantom{a}\smash{\mathbf {#1}}}} \)
To account for the origin of life on our earth requires solving several problems:
- How the organic molecules that define life, e.g. amino acids, nucleotides, were created.
- How these were assembled into macromolecules, e.g. proteins and nucleic acids, - a process requiring catalysts.
- How these were able to reproduce themselves.
- How these were assembled into a system delimited from its surroundings (i.e., a cell).
A number of theories address each of these problems. As for the first problem, four scenarios have been proposed. Organic molecules:
- were synthesized from inorganic compounds in the atmosphere,
- rained down on earth from outer space,
- were synthesized at hydrothermal vents on the ocean floor,
- were synthesized when comets or asteroids struck the early earth.
Scenario 1: Miller's Experiment
Stanley Miller, a graduate student in biochemistry, built the apparatus shown in Figure \(\PageIndex{1}\). He filled it with water (H 2 O), methane (CH 4 ), ammonia (NH 3 ) and hydrogen (H 2 ), but no oxygen. He hypothesized that this mixture resembled the atmosphere of the early earth. The mixture was kept circulating by continuously boiling and then condensing the water. The gases passed through a chamber containing two electrodes with a spark passing between them.
At the end of a week, Miller used paper chromatography to show that the flask now contained several amino acids as well as some other organic molecules. However, it is now thought that the atmosphere of the early earth was not rich in methane and ammonia - essential ingredients in Miller's experiments. In the years since Miller's work, many variants of his procedure have been tried. Virtually all the small molecules that are associated with life have been formed:
- 17 of the 20 amino acids used in protein synthesis, and all the purines and pyrimidines used in nucleic acid synthesis.
- But abiotic synthesis of ribose - and thus of nucleotides - has been much more difficult. However, success in synthesizing pyrimidine ribonucleotides under conditions that might have existed in the early earth was reported in the 14 May 2009 issue of Nature .
- And in 2015, chemists in Cambridge England led by John Sutherland reported that they had been able to synthesize precursors of 12 of the 20 amino acids and two (of the four) ribonucleotides used by life as well as glycerol-1-phosphate, a precursor of lipids. They created all of these molecules using only hydrogen cyanide (HCN) and hydrogen sulfide (H 2 S) irradiated with ultraviolet light in the presence of mineral catalysts.
Scenario 2: Molecules from Outer Space
Astronomers, using infrared spectroscopy, have identified a variety of organic molecules in interstellar space, including methane (CH 4 ), methanol (CH 3 OH), formaldehyde (HCHO), cyanoacetylene (HC 3 N) (which in spark-discharge experiments is a precursor to the pyrimidine cytosine ), polycyclic aromatic hydrocarbonsas well as such inorganic building blocks as carbon dioxide (CO 2 ), carbon monoxide (CO), ammonia (NH 3 ), hydrogen sulfide (H 2 S), and hydrogen cyanide (HCN).
There have been several reports of producing amino acids and other organic molecules in laboratories by taking a mixture of molecules known to be present in interstellar space such as ammonia (NH 3 ), carbon monoxide (CO), methanol (CH 3 OH) and water (H 2 O), hydrogen cyanide (HCN) and exposing it to a temperature close to that of space (near absolute zero) and intense ultraviolet (UV) radiation. Whether or not the molecules that formed terrestrial life arrived here from space, there is little doubt that organic matter continuously rains down on the earth (estimated at 30 tons per day).
Alternatively, organic molecules can be transport to Earth via meteorites as demonstrated with the Murchison Meteorite that that fell near Murchison, Australia on 28 September 1969. This meteorite turned out to contain a variety of organic molecules including: purines and pyrimidines, polyols - compounds with hydroxyl groups on a backbone of 3 to 6 carbons such as glycerol and glyceric acid (sugars are polyols) and the amino acids listed in Table \(\PageIndex{1}\). The amino acids and their relative proportions were quite similar to the products formed in Miller's experiments.
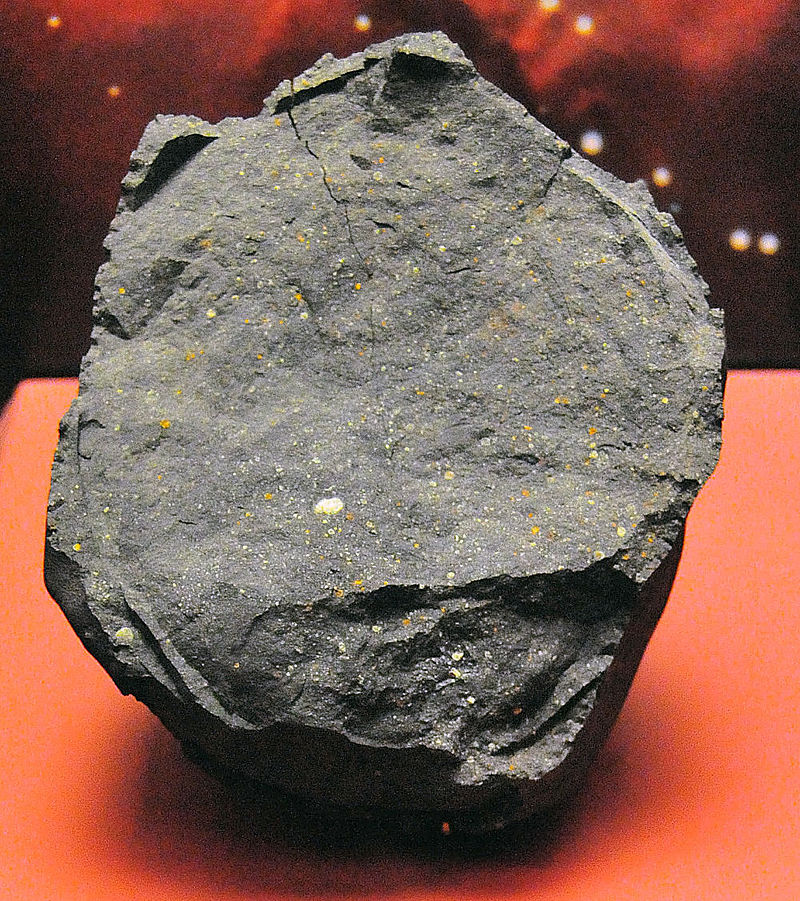
Murchison meteorite at the The National Museum of Natural History (Washington). (CC SA-BY 3.0; :Basilicofresco ).
Contamination?
The question is if these molecules identified in the Murchison meteorite were simply terrestrial contaminants that got into the meteorite after it fell to earth? Probably not:
- Some of the samples were collected on the same day it fell and subsequently handled with great care to avoid contamination.
- The polyols contained the isotopes carbon-13 and hydrogen-2 (deuterium) in greater amounts than found here on earth.
- The samples lacked certain amino acids that are found in all earthly proteins.
- Only L amino acids occur in earthly proteins, but the amino acids in the meteorite contain both D and L forms (although L forms were slightly more prevalent).
Scenario 3: Deep-Sea Hydrothermal Vents
Some deep-sea hydrothermal vents discharge copious amounts of hydrogen, hydrogen sulfide, and carbon dioxide at temperatures around 100°C. (These are not "black smokers".) These gases bubble up through chambers rich in iron sulfides (FeS, FeS 2 ). These can catalyze the formation of simple organic molecules like acetate. (And life today depends on enzymes that have Fe and S atoms in their active sites.)
Scenario 4: Laboratory Synthesis of Nucleobases Under Conditions Mimicking the Impact of Asteroids or Comets on the Early Earth
Researchers in the Czech Republic reported in 2014 that they had succeeded in the abiotic synthesis of adenine (A), guanine (G), cytosine (C), and uracil (U) — the four bases found in RNA (an RNA beginning?) and three of the four found in DNA. They achieved this by bombarding a mixture of formamide and clay with powerful laser pulses that mimicked the temperature and pressure expected when a large meteorite strikes the earth. Formamide is a simple substance, CH 3 NO, thought to have been abundant on the early earth and containing the four elements fundamental to all life.
Assembling Polymers
Another problem is how polymers - the basis of life itself - could be assembled.
- In solution, hydrolysis of a growing polymer would soon limit the size it could reach.
- Abiotic synthesis produces a mixture of L and D enantiomers. Each inhibits the polymerization of the other. (So, for example, the presence of D amino acids inhibits the polymerization of L amino acids (the ones that make up proteins here on earth).
This has led to a theory that early polymers were assembled on solid, mineral surfaces that protected them from degradation, and in the laboratory polypeptides and polynucleotides (RNA molecules) containing about ~50 units have been synthesized on mineral (e.g., clay) surfaces.
An RNA Beginning?
All metabolism depends on enzymes and, until recently, every enzyme has turned out to be a protein. But proteins are synthesized from information encoded in DNA and translated into mRNA. So here is a chicken-and-egg dilemma. The synthesis of DNA and RNA requires proteins. So proteins cannot be made without nucleic acids and nucleic acids cannot be made without proteins. The discovery that certain RNA molecules have enzymatic activity provides a possible solution. These RNA molecules — called ribozymes — incorporate both the features required of life: storage of information and the ability to act as catalysts.
While no ribozyme in nature has yet been found that can replicate itself, ribozymes have been synthesized in the laboratory that can catalyze the assembly of short oligonucleotides into exact complements of themselves. The ribozyme serves as both the template on which short lengths of RNA ("oligonucleotides" are assembled following the rules of base pairing and the catalyst for covalently linking these oligonucleotides.
In principal, the minimal functions of life might have begun with RNA and only later did proteins take over the catalytic machinery of metabolism and DNA take over as the repository of the genetic code. Several other bits of evidence support this notion of an original "RNA world":
- In the cell, all deoxyribonucleotides are synthesized from ribonucleotide precursors.
- Many bacteria control the transcription and/or translation of certain genes with RNA molecules, not protein molecules.
Reproduction?
Perhaps the earliest form of reproduction was a simple fission of the growing aggregate into two parts - each with identical metabolic and genetic systems intact.
The First Cell?
To function, the machinery of life must be separated from its surroundings - some form of extracellular fluid (ECF). This function is provided by the plasma membrane. Today's plasma membranes are made of a double layer of phospholipids. They are only permeable to small, uncharged molecules like H 2 O, CO 2 , and O 2 . Specialized transmembrane transporters are needed for ions, hydrophilic, and charged organic molecules (e.g., amino acids and nucleotides) to pass into and out of the cell.
However, the same Szostak lab that produced the finding described above reported in the 3 July 2008 issue of Nature that fatty acids, fatty alcohols, and monoglycerides - all molecules that can be synthesized under prebiotic conditions - can also form lipid bilayers and these can spontaneously assemble into enclosed vesicles.
Unlike phospholipid vesicles, these
- admit from the external medium charged molecules like nucleotides
- admit from the external medium hydrophilic molecules like ribose
- grow by self-assembly
- are impermeable to, and thus retain, polymers like oligonucleotides.
These workers loaded their synthetic vesicles with a short single strand of deoxycytidine (dC) structured to provide a template for its replication. When the vesicles were placed in a medium containing (chemically modified) dG, these nucleotides entered the vesicles and assembled into a strand of Gs complementary to the template strand of Cs. Here, then, is a simple system that is a plausible model for the creation of the first cells from the primeval "soup" of organic molecules.
From Unicellular to Multicellular Organisms
This transition is probably the easiest to understand.

Several colonial flagellated green algae provide a clue. These species are called colonial because they are made up simply of clusters of independent cells. If a single cell of Gonium , Pandorina , or Eudorina is isolated from the rest of the colony, it will swim away looking quite like a Chlamydomonas cell. Then, as it undergoes mitosis, it will form a new colony with the characteristic number of cells in that colony.
(The figures are not drawn to scale. Their sizes range from Chlamydomonas which is about 10 µm in diameter - little larger than a human red blood cell - to Volvox whose sphere is some 350 µm in diameter - visible to the naked eye.)
The situation in Pleodorina and Volvox is different. In these organisms, some of the cells of the colony (most in Volvox) are not able to live independently. If a nonreproductive cell is isolated from a Volvox colony, it will fail to reproduce itself by mitosis and eventually will die. What has happened? In some way, as yet unclear, Volvox has crossed the line separating simple colonial organisms from truly multicellular ones. Unlike Gonium, Volvox cannot be considered simply a colony of individual cells. It is a single organism whose cells have lost their ability to live independently. If a sufficient number of them become damaged, the entire sphere of cells will die.
What has Volvox gained? In giving up their independence, the cells of Volvox have become specialists. No longer does every cell carry out all of life's functions (as in colonial forms); instead certain cells specialize to carry out certain functions while leaving other functions to other specialists. In Volvox this process goes no further than having certain cells specialize for reproduction while others, unable to reproduce themselves, fulfill the needs for photosynthesis and locomotion.
In more complex multicellular organisms, the degree of specialization is carried much further. Each cell has one or two precise functions to carry out. It depends on other cells to carry out all the other functions needed to maintain the life of the organism and thus its own.
The specialization and division of labor among cells is the outcome of their history of differentiation. One of the great problems in biology is how differentiation arises among cells, all of which having arisen by mitosis, share the same genes.
The genomes of both Chlamydomonas and Volvox have been sequenced. Although one is unicellular, the other multicellular, they have not only about the same number of protein-encoding genes (14,516 in Chlamydomonas, 14,520 in Volvox) but most of these are homologous. Volvox has only 58 genes that have no relatives in Chlamydomonas and even fewer unique mRNAs.
At one time, many of us would have expected that a multicellular organism like Volvox with its differentiated cells and complex life cycle would have had many more genes than a single-celled organism like Chlamydomonas. But that turns out not to be the case.
How to explain this apparent paradox? My guess is that just as we have seen in the evolution of animals, we are seeing here that the evolution of organismic complexity is not so much a matter of the evolution of new genes but rather the evolution of changes in the control elements (promoters and enhancers) that dictate how and where the basic tool kit of eukaryotic genes will be expressed .
The evidence is compelling that all these organisms are close relatives; that is, belong to the same clade. They illustrate how colonial forms could arise from unicellular ones and multicellular forms from colonial ones.
The Last Universal Common Ancestor (LUCA)?
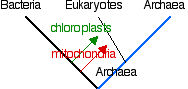
Creating Life?
When I headed off to college (in 1949), I wrote an essay speculating on the possibility that some day we would be able to create a living organism from nonliving ingredients. By the time I finished my formal studies in biology — having learned of the incredible complexity of even the simplest organism — I concluded that such a feat could never be accomplished.
Now I'm not so sure.
Several recent advances suggest that we may be getting close to creating life. (But note that these examples represent laboratory manipulations that do not necessarily reflect what may have happened when life first appeared.)
- The ability to created membrane-enclosed vesicles that can take in small molecules and assemble them into polymers which remain within the "cell".
- The ability to assemble functional ribosomes — the structures that convert the information encoded in the genome into the proteins that run life — from their components.
Could this be placed in the cytoplasm of a living cell and run it?
The same team showed in the previous year (see Science 3 August 2007) that they could insert an entire chromosome from one species of mycoplasma into the cytoplasm of a related species and, in due course, the recipient lost its own chromosome (perhaps destroyed by restriction enzymes encoded by the donor chromosome) and began expressing the phenotype of the donor. In short, they had changed one species into another. But the donor chromosome was made by the donor bacterium, not synthesized in the laboratory. However, there should be no serious obstacle to achieving the same genome transplantation with a chemically-synthesized chromosome.
They've done it! The same team reported on 20 May 2010 in the online Science Express that they had successfully transplanted a completely synthetic genome — based on that of Mycoplasma mycoides — into the related species Mycoplasma capricolum . The recipient strain grew well and soon acquired the phenotype of the M. mycoides donor.
Their procedure:
- Chemically synthesize 69- to 79-nt oligonucleotides representing all the stretches of the known chromosome 9 sequence (which contains 316,617 base pairs) except for certain sequences such as transposons, many introns, and transfer RNA genes. In addition new, non-native, sequences such as loxP sites were included to aid future manipulations of the genome.
- Stitch these together into blocks of ~750 base pairs. This step was done in vitro by undergraduates enrolled in the "Build A Genome" class at Johns Hopkins.
- Introduce these into yeast cells which ligated them into stretches of DNA containing 2–4 thousand base pairs.
- Introduce these stepwise into yeast cells so that they replace the equivalent portions of the native chromosome.
- The result: a strain of yeast that grows just as well with its new artificial chromosome (now containing only 272,871 base pairs) as it did before.
The Role of Meteorite Impacts in the Origin of Life
Affiliations.
- 1 Institute for Earth and Space Exploration, University of Western Ontario, London, Canada.
- 2 Department of Earth Sciences, University of Western Ontario, London, Canada.
- 3 UK Centre for Astrobiology, School of Physics and Astronomy, University of Edinburgh, Edinburgh, UK.
- 4 Department of Biology, Georgetown University, Washington, DC, USA.
- 5 Division of Geological and Planetary Sciences, California Institute of Technology, Pasadena, California, USA.
- 6 Department of Earth Sciences, University of Southern California, Los Angeles, California, USA.
- PMID: 32876492
- PMCID: PMC7499892
- DOI: 10.1089/ast.2019.2203
The conditions, timing, and setting for the origin of life on Earth and whether life exists elsewhere in our solar system and beyond represent some of the most fundamental scientific questions of our time. Although the bombardment of planets and satellites by asteroids and comets has long been viewed as a destructive process that would have presented a barrier to the emergence of life and frustrated or extinguished life, we provide a comprehensive synthesis of data and observations on the beneficial role of impacts in a wide range of prebiotic and biological processes. In the context of previously proposed environments for the origin of life on Earth, we discuss how meteorite impacts can generate both subaerial and submarine hydrothermal vents, abundant hydrothermal-sedimentary settings, and impact analogues for volcanic pumice rafts and splash pools. Impact events can also deliver and/or generate many of the necessary chemical ingredients for life and catalytic substrates such as clays as well. The role that impact cratering plays in fracturing planetary crusts and its effects on deep subsurface habitats for life are also discussed. In summary, we propose that meteorite impact events are a fundamental geobiological process in planetary evolution that played an important role in the origin of life on Earth. We conclude with the recommendation that impact craters should be considered prime sites in the search for evidence of past life on Mars. Furthermore, unlike other geological processes such as volcanism or plate tectonics, impact cratering is ubiquitous on planetary bodies throughout the Universe and is independent of size, composition, and distance from the host star. Impact events thus provide a mechanism with the potential to generate habitable planets, moons, and asteroids throughout the Solar System and beyond.
Keywords: Crater lakes; Geobiology; Hadean environment; Hydrothermal systems; Impact craters; Lithophytic habitats; Origin of life.
Publication types
- Research Support, Non-U.S. Gov't
- Earth, Planet*
- Evolution, Chemical*
- Extraterrestrial Environment / chemistry*
- Geological Phenomena
- Meteoroids*
- Origin of Life*
- Reference work entry
- First Online: 01 January 2018
- Cite this reference work entry
- Michael K. Weisberg 5
Part of the book series: Encyclopedia of Earth Sciences Series ((EESS))
272 Accesses
1 Citations
Meteorites are rock fragments dislodged from a celestial body, launched into interplanetary space, which pass through the Earth’s atmosphere and land on the surface. However, rocks that similarly fell to the surface of the Moon and Mars (or any other planetary body) are also meteorites. Additionally, a rock launched from a planetary body and falls back to that same body is also considered a meteorite. A comprehensive definition (from Rubin and Grossman 2014 ) is a meteorite is a natural, solid object larger than 10 μm in size, derived from a celestial body, that was transported by natural means from the body on which it formed to a region outside the dominant gravitational influence of that body and that later collided with a natural or artificial body larger than itself (even if it is the same body from which it was launched). Weathering and other secondary processes do not affect an object’s status as a meteorite as long as something recognizable remains of its original...
This is a preview of subscription content, log in via an institution to check access.
Access this chapter
- Available as PDF
- Read on any device
- Instant download
- Own it forever
- Available as EPUB and PDF
- Durable hardcover edition
- Dispatched in 3 to 5 business days
- Free shipping worldwide - see info
Tax calculation will be finalised at checkout
Purchases are for personal use only
Institutional subscriptions
Benedix GK, McCoy TJ, Keil K, Boggard DD, Garrison DH (1998) A petrologic and isotopic study of winonaites: evidence for early partial melting, brecciation, and metamorphism. Geochim Cosmochim Acta 62:2535–2553
Article Google Scholar
Benedix GK, McCoy TJ, Keil K, Love SG (2000) A petrologic study of the IAB iron meteorites: constraints on the formation of the IAB-winonaite parent body. Meteorit Planet Sci 35:1127–1141
Binzel RP, Xu S (1993) Chips off of asteroid 4 Vesta: evidence for the parent body of basaltic achondrite meteorites. Science 260:186–191
Boesenberg JS, Davis AM, Prinz M, Weisberg MK, Clayton RN, Mayeda TK (2000) The pyroxene pallasites, vermillion and Yamato 8451: not quite a couple. Meteorit Planet Sci 35:757–769
Bogard DD, Johnson P (1983) Martian gases in an Antarctic meteorite. Science 221:651–654
Bouvier A, Wadhwa M (2010) The age of the solar system redefined by the oldest Pb–Pb age of a meteoritic inclusion. Nat Geosci 3:637–641
Choi BG, Ouyang X, Wasson JT (1995) Classification and origin of IAB and IICD iron meteorites. Geochim Cosmochim Acta 59:593–612
Clayton RN (2003) Oxygen isotopes in meteorites. In: Davis AM (ed) Treatise on Geochemistry 1, Meteorites, Comets, and Planets. Elsevier-Pergamon, Oxford, pp 129–142
Google Scholar
Clayton RN, Mayeda TK (1984) Oxygen isotope compositions of enstatite chondrites and aubrites. J Geophys Res 89:C245–C249. Proceeding of the fifteenth Lunar and Planetary Science Conference
Clayton RN, Mayeda TK (1988) Formation of ureilites by nebular processes. Geochim Cosmochim Acta 52:1313–1318
Clayton RN, Mayeda TK (1996) Oxygen-isotope studies of achondrites. Geochim Cosmochim Acta 60:1999–2018
Clayton RN, Mayeda TK (1999) Oxygen isotope studies of carbonaceous chondrites. Geochim Cosmochim Acta 63:2089–2104
Delaney JS, Prinz M, Takeda H (1984) The polymict eucrites. J Geophys Res Supplement, 89:C251–C288. Proceedings of the 15th Lunar and Planetary Science Conference
Fitoussi C, Bourdon B (2012) Silicon isotope evidence against an enstatite chondrite earth. Science 335:1477–1480
Floran RJ, Caulfield JBD, Harlow GE, Prinz M (1978) Impact origin for the Simondium, Pinnaroo, and Hainholz mesosiderites: implications for impact processes beyond the earth-moon system. Proc Lunar Planet Sci Conf 9:1083–1114
Grevesse N, Sauval AJ (1998) Standard solar composition. Space Sci Rev 85:161–174
Hewins RH (1983) Impact versus internal origins for mesosiderites. J Geophys Res 88:B257–B266
Javoy M (1995) The integral enstatite chondrite model of the earth. Geophys Res Lett 22:2219–2222
Kallemeyn GW, Wasson JT (1981) The compositional classification of chondrites: I the carbonaceous chondrite groups. Geochim Cosmochim Acta 45:1217–1230
Kallemeyn GW, Wasson JT (1982) The compositional classification of chondrites: III Ungrouped carbonaceous chondrites. Geochim Cosmochim Acta 46:2217–2228
Kallemeyn GW, Boynton WV, Willis J, Wasson JT (1978) Formation of the Bencubbin polymict meteoritic breccia. Geochim Cosmochim Acta 42:507–515
Kallemeyn GW, Rubin AE, Wang D, Wasson JT (1989) Ordinary chondrites: bulk compositions, classification, lithophile-element fractionations, and composition-petrographic type relationships. Geochim Cosmochim Acta 53:2747–2767
Kallemeyn GW, Rubin AE, Wasson JT (1991) The compositional classification of chondrites: V. The Karoonda (CK) group of carbonaceous chondrites. Geochim Cosmochim Acta 55:881–892
Kallemeyn GW, Rubin AE, Wasson JT (1994) The compositional classification of chondrites: VI The CR carbonaceous chondrite group. Geochim Cosmochim Acta 58:2873–2888
Kallemeyn GW, Rubin AE, Wasson JT (1996) The compositional classification of chondrites: VII the R chondrite group. Geochim Cosmochim Acta 60:2243–2256
Keil K (1969) Mineralogical and chemical relationships among enstatite chondrites. J Geophys Res 73:6945–6976
Keil K (1989) Enstatite meteorites and their parent bodies. Meteoritics 24:195–208
Kimura M, Tsuchiayama A, Fukuoka T, Iimura Y (1992) Antarctic primitive achondrites, Yamato-74025, −75300, and −75305: their mineralogy, thermal history, and the relevance to winonaites. Proc NIPR Symp Antarct Meteorites 5:165–190
Kracher A (1982) Crystallization of a S-saturated -melt, and the origin of the iron meteorite groups IAB and IIICD. Geophys Res Lett 9:412–415
McCord TB, Adams JB, Johnson TV (1970) Asteroid Vesta: spectral reflectivity and compositional implications. Science 168:1445–1447
McCoy TJ, Scott ERD, Haack H (1993) Genesis of the IIICD iron meteorites: evidence from silicate-bearing inclusions. Meteoritics 28:552–560
McCoy TJ, Keil K, Clayton RN, Mayeda TK, Bogard DD, Garrison DH, Huss GR, Hutcheon ID, Wieler R (1996) A petrologic, chemical and isotopic study of monument draw and comparison with other acapulcoites: evidence for formation by incipient partial melting. Geochim Cosmochim Acta 60:2681–2708
McKay DS, Gibson EK, Thomas-Keprta VH, Romanek CS, Clement SJ, Chillier XDF, Maechling CR, Zare RN (1996) Search for past life on Mars: possible relic biogenic activity in martian meteorite ALH84001. Science 273:924–930
McSween HY Jr (2002) The rocks of Mars, from far and near. Meteorit Planet Sci 37:7–25
McSween HY Jr, Treiman AH (1998) Martian meteorites. In Planetary Materials (J.J. Papike ed.) Rev Mineral 36:6-1–6-53
Mittlefehldt DW (1994) ALH84001, a cumulate orthopyroxenite member of the martian meteorite clan. Meteoritics 29:214–221
Mittlefehldt DW, Lindstrom MM (1990) Geochemistry and genesis of the Angrites. Geochim Cosmochim Acta 54:3209–3218
Mittlefehldt DW, McCoy TJ, Goodrich CA, Kracher A (1998) Non-chondritic meteorites from asteroidal bodies in planetary materials (J.J. Papike, ed.) Rev Mineral 36:4.1–4.495
Nagahara H (1992) Yamato-8002: partial melting residue on the “unique” chondrite parent body. Proc NIPR Symp Antarct Meteorit 5:191–223
Nyquist LE, Bogard DD, Shih C-Y, Greshake A, Stöffler D, Eugster O (2001) Ages and geologic histories of martian meteorites. Space Sci Rev 96:105–164
Palme H, Jones A (2003) Solar abundances of the elements. In: Davis AM (ed) Treatise on geochemistry 1, meteorites, comets, and planets. Elsevier-Pergamon, Oxford, pp 41–61
Paniello R, Day JMD, Moynier F (2012) Zn isotopic evidence for the origin of the moon. Nature 490:376–379
Papike JJ, Ryder G, Shearer CK (1998) Lunar samples. In planetary materials (J.J. Papike. Ed.) Rev Mineral 36:5.1–5.234
Rubin AE, Grossman JN (2014) Meteorite and meteoroid: a new comprehensive definition. Meteorit Planet Sci 45:114–122
Savage PS, Moynier F (2013) Silicon isotopic variation in enstatite meteorites: clues to their origin and earth-forming material. Earth Planet Sci Lett 361:487–496
Scott ERD (1972) Chemical fractionation in iron meteorites and its interpretation. Geochim. Cosmochim. Acta 36:1205–1236
Scott ERD, Wasson JT (1975) Classification and properties of iron meteorites. Rev Geophys Space Phys 13:527–546
Scott ERD, Haack H, Love SG (2001) Formation of mesosiderites by fragmentation and reaccretion of a large differentiated asteroid. Meteorit Planet Sci 36:869–881
Sears DWG, Kallemeyn GW, Wasson JT (1982) The compositional classification of chondrites: II The enstatite chondrite groups. Geochim Cosmochim Acta 46:597–608
Warren PH (2011) Stable-isotopic anomalies and the accretionary assemblage of the earth and Mars: a subordinate role for carbonaceous chondrites. Earth Planet Sci Lett 311:93–100
Wasson JT, Kallemeyn GW (1988) Composition of chondrites. Philos Trans R Soc Lond A325:535–544
Watters TR, Prinz M (1979) Aubrites: their origin and relationship to enstatite chondrites. Proc Lunar Planet Sci Conf 10:1073–1093
Weisberg MK, McCoy TJ, Krot AE (2006) Systematics and evaluation of meteorite classification. In: Lauretta D, McSween HY Jr (eds) Meteorites and the early solar system II. University of Arizona Press, Tucson, pp 19–52
Weisberg MK, Ebel DS, Nagashima D, Kita N, Humayun M (2015) Petrology and geochemistry of chondrules and metal in NWA 5492 and GRO 95551: a new type of metal-rich chondrite. Geochim Cosmochim Acta 167:269–285
Weiss BP, Gattacceca J, Sabine S, Rochette P, Christensen UR (2010) Paleomagnetic record of meteorites and early planetesimal differentiation. Space Sci Rev 152:341–390
Wheelock MM, Keil K, Floss C, Taylor GJ, Crozaz G (1994) REE geochemistry of oldhamite-dominated clasts from the Norton County aubrite: igneous origin of oldhamite. Geochim Cosmochim Acta 58:449–458
Yanai K (1994) Angrite Asuka-881371: preliminary examination of a unique meteorite in the Japanese collection of Antarctic meteorites. Proc NIPR Symp Antarct Meteorites 7:30–41
Download references
Author information
Authors and affiliations.
Department of Physical Sciences, Earth and Planetary Sciences, Kingsborough Community College and Graduate Center, City University of New York, New York, USA
Michael K. Weisberg
You can also search for this author in PubMed Google Scholar
Corresponding author
Correspondence to Michael K. Weisberg .
Editor information
Editors and affiliations.
Cornell University, Ithaca, NY, USA
William M. White
Rights and permissions
Reprints and permissions
Copyright information
© 2018 Springer International Publishing AG, part of Springer Nature
About this entry
Cite this entry.
Weisberg, M.K. (2018). Meteorites. In: White, W.M. (eds) Encyclopedia of Geochemistry. Encyclopedia of Earth Sciences Series. Springer, Cham. https://doi.org/10.1007/978-3-319-39312-4_331
Download citation
DOI : https://doi.org/10.1007/978-3-319-39312-4_331
Published : 18 July 2018
Publisher Name : Springer, Cham
Print ISBN : 978-3-319-39311-7
Online ISBN : 978-3-319-39312-4
eBook Packages : Earth and Environmental Science Reference Module Physical and Materials Science Reference Module Earth and Environmental Sciences
Share this entry
Anyone you share the following link with will be able to read this content:
Sorry, a shareable link is not currently available for this article.
Provided by the Springer Nature SharedIt content-sharing initiative
- Publish with us
Policies and ethics
- Find a journal
- Track your research
Thank you for visiting nature.com. You are using a browser version with limited support for CSS. To obtain the best experience, we recommend you use a more up to date browser (or turn off compatibility mode in Internet Explorer). In the meantime, to ensure continued support, we are displaying the site without styles and JavaScript.
- View all journals
- Explore content
- About the journal
- Publish with us
- Sign up for alerts
- BOOK REVIEW
- 25 January 2021
Meteorites: ‘a story written across light years down the barrel of a microscope’
- Meenakshi Wadhwa 0
Meenakshi Wadhwa is director and professor in the School of Earth and Space Exploration at Arizona State University in Tempe. Asteroid 8356 Wadhwa is named in recognition of her contributions to meteoritics and planetary science.
You can also search for this author in PubMed Google Scholar
Meteorite: The Stones From Outer Space That Made Our World Tim Gregory John Murray (2020)
Access options
Access Nature and 54 other Nature Portfolio journals
Get Nature+, our best-value online-access subscription
24,99 € / 30 days
cancel any time
Subscribe to this journal
Receive 51 print issues and online access
185,98 € per year
only 3,65 € per issue
Rent or buy this article
Prices vary by article type
Prices may be subject to local taxes which are calculated during checkout
Nature 589 , 510-511 (2021)
doi: https://doi.org/10.1038/d41586-021-00188-8
Related Articles
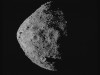
- Astronomy and astrophysics
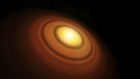
Is that a giant sandwich? No, it’s the biggest protoplanetary disk in the sky
Research Highlight 23 MAY 24
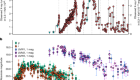
Lense–Thirring precession after a supermassive black hole disrupts a star
Article 22 MAY 24
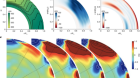
The solar dynamo begins near the surface
Stereospecific alkenylidene homologation of organoboronates by SNV reaction
Article 23 MAY 24
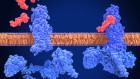
Two receptors are better than one for AI-designed obesity drugs
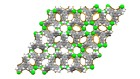
Superstar porous materials get salty thanks to computer simulations
News 23 MAY 24
Professor, Division Director, Translational and Clinical Pharmacology
Cincinnati Children’s seeks a director of the Division of Translational and Clinical Pharmacology.
Cincinnati, Ohio
Cincinnati Children's Hospital & Medical Center
Data Analyst for Gene Regulation as an Academic Functional Specialist
The Rheinische Friedrich-Wilhelms-Universität Bonn is an international research university with a broad spectrum of subjects. With 200 years of his...
53113, Bonn (DE)
Rheinische Friedrich-Wilhelms-Universität
Recruitment of Global Talent at the Institute of Zoology, Chinese Academy of Sciences (IOZ, CAS)
The Institute of Zoology (IOZ), Chinese Academy of Sciences (CAS), is seeking global talents around the world.
Beijing, China
Institute of Zoology, Chinese Academy of Sciences (IOZ, CAS)
Full Professorship (W3) in “Organic Environmental Geochemistry (f/m/d)
The Institute of Earth Sciences within the Faculty of Chemistry and Earth Sciences at Heidelberg University invites applications for a FULL PROFE...
Heidelberg, Brandenburg (DE)
Universität Heidelberg
Postdoctoral scholarship in Structural biology of neurodegeneration
A 2-year fellowship in multidisciplinary project combining molecular, structural and cell biology approaches to understand neurodegenerative disease
Umeå, Sweden
Umeå University
Sign up for the Nature Briefing newsletter — what matters in science, free to your inbox daily.
Quick links
- Explore articles by subject
- Guide to authors
- Editorial policies
Accept cookies?
We use cook ies to give you the best online experience and to show personalised content and marketing. We use them to improve our website and content as well as to tailor our digital advertising on third-party platforms. You can change your preferences at any time.
Popular search terms:
- British wildlife
- Wildlife Photographer of the Year
- Explore the Museum
Anthropocene
British Wildlife
Collections
Human evolution
What on Earth?
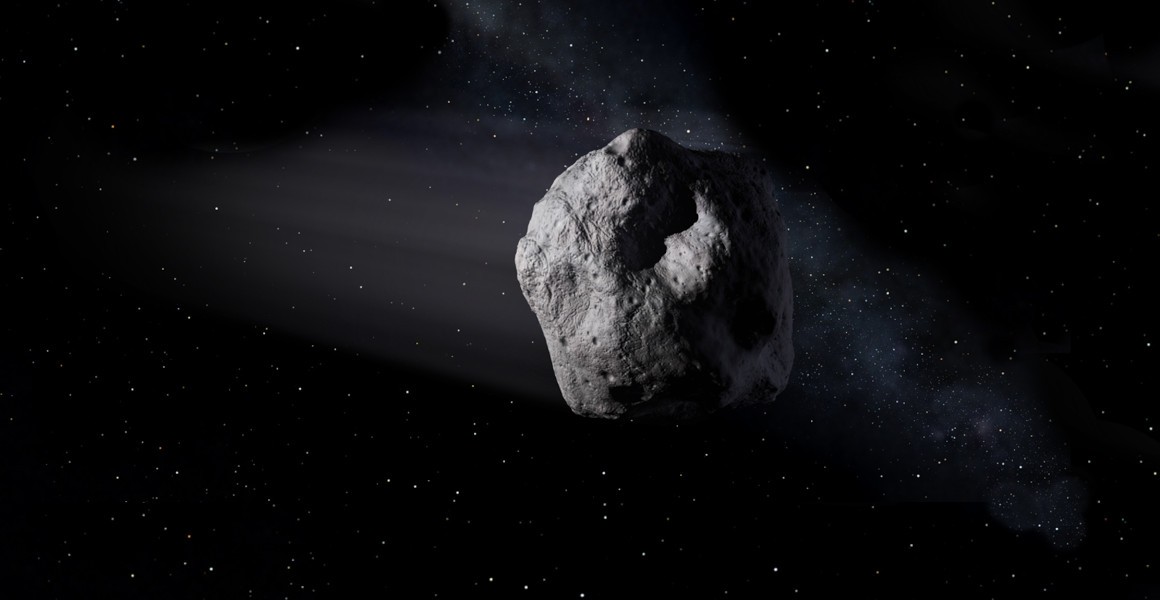
Asteroids could have allowed the formation of DNA bases in space before falling as meteorites to Earth. Image © NASA
During Beta testing articles may only be saved for seven days.
Create a list of articles to read later. You will be able to access your list from any article in Discover.
You don't have any saved articles.
Meteorites could have brought DNA precursors to Earth
Meteorites could have been responsible for delivering the basis of life's genetic code.
Analyses of three meteorites suggest that nucleobases, the crucial components of DNA, could have formed in space and then fallen to Earth to provide the raw material for the origin of life itself.
Could meteorites have brought the building blocks of life to Earth?
A new study, published in Nature Communications , suggests this could be the case. Analysing a group of meteorites known to be rich in organic materials, the study is one of the first to demonstrate that both types of nucleobase, the compounds that encode genetic information in DNA, could have originally had an extraterrestrial origin.
After the formation of nucleobases in outer space, the meteorites would have been able to deliver the molecules to Earth, later becoming the basis of all genetic coding for life.
Dr Helena Bates , who researches asteroids at the Museum and was not involved in the study, says, 'During the formation of Earth, many common elements and compounds with low boiling points, known as volatiles, would not have been present. We believe that many of these, such as water, were delivered afterwards by meteorites, which may have also delivered organic molecules.
'This paper finds that nucleobases were among those organic compounds potentially delivered by meteorites, and suggests that these molecules may have contributed to the emergence of genetic material on Earth.
'It's very strange to think that the molecules which make up DNA can be found in rocks from space. It suggests that these molecules are all over the place, which has a lot of implications when it comes to considering the potential distribution of life within our Solar System.'
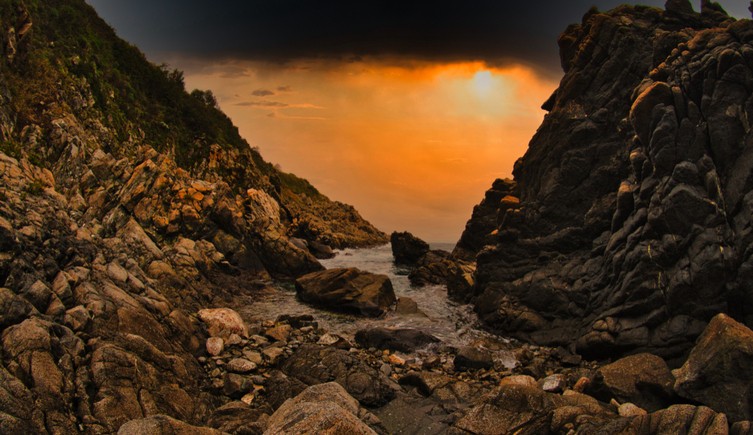
Before the origin of life on Earth, a variety of inorganic processes gave rise for the right conditions for it to come about. Image © Antonio Arico/Shutterstock
What conditions were needed for the origin of life?
There are many different theories for what conditions were needed for life to form on Earth. One of the most famous is the primordial soup hypothesis, which suggests that intense ultraviolet radiation and lightning on the early Earth provided the energy for chemical reactions between compounds such as water, ammonia and methane to begin.
The theory suggests these reactions then led to the creation of molecules such as DNA and RNA, which subsequently became part of a self-replicating system which would ultimately become life. Experiments have confirmed that this theory might work, with subsequent analysis showing that more than 20 amino acids crucial for life were synthesised.
However, there are some gaps between the conditions simulated in these experiments and what we now understand about the early evolution of Earth's atmosphere. The challenges of the primordial soup hypothesis have led some scientists to look elsewhere for how the earliest molecules formed.
The bottom of the ocean presents some promising candidates, with alkaline vents on the ocean floor being rich in the compounds needed for the formation of nucleic acids.
Other theories include reactions involving ice and glaciers, radioactive beaches, and meteorites bringing these materials to Earth. The latter are already known to have played a crucial part in the formation of life as we know it, with a type known as the carbonaceous chondrites believed to have delivered most of the planet's water.
Meteorites also contain a variety of other organic molecules. Charlotte Bays is a PhD student at the Museum who was not involved with the study but researches these organic compounds. She says, 'The intricacies of how these molecules form in the interstellar medium is still widely debated, as there are a number of different ways in which they could be synthesised.
'What's interesting about this paper is that it finds nucleobases, which make up the structure of RNA and DNA, in these meteorites. While guanine and adenine have previously been identified, the discovery of cytosine, uracil and thymine complete the set needed for forming base pairs.
'The occurrence of these kind of complex molecules that we're seeing in these meteorites get around some of the issues of them forming on Earth, as the delivery of these initial building blocks would have paved the way for critical biorelevant molecules such as DNA and RNA to form later.'
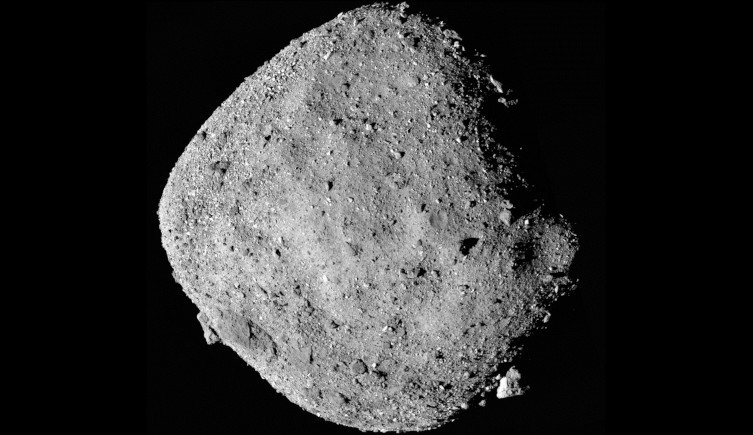
Asteroids such as Bennu may be the parent bodies of carbonaceous chondrite meteorites. Image © NASA's Marshall Space Flight Center, licensed under CC BY-NC 2.0 via Flickr .
Which DNA precursors were found in the meteorites?
The study, a collaboration between Japanese and American scientists, studied three carbonaceous meteorites which fell in Murchison, Australia; Lake Murray, USA; and Tagish Lake, Canada.
Samples were taken from these meteorites and ground into a fine powder, allowing the organic components of each meteorite to be extracted. All five of the purines and pyrimidines which make up DNA and RNA were detected in the meteorites at concentrations similar to experiments replicating the conditions of the early universe.
The researchers ruled out that these compounds were the result of contamination after the meteorites landed on Earth, as the nucleobase concentrations were different from the soil found in the areas they landed. The typical precursors for their biological formation were also mostly absent, suggesting the molecules formed in a way unlike those on Earth.
The next step is to analyse samples of asteroids which may have been similar to the parent bodies of these meteorites, potentially allowing the researchers to confirm their findings.
Previous missions have already detected organic molecules on Itokawa , a siliceous asteroid, while missions to the asteroids Ryugu and Bennu are expected to be analysed in the coming years. This paper adds to this work, demonstrating the presence of nucleobases on other asteroid types.
'Two of the meteorites are thought to come from B- and C-type asteroids, like Bennu and Ryugu, which are common in the Solar System,' Charlotte says. 'However, the Tagish Lake meteorite has been suggested to come from a D-type asteroid , which are rarer.
'As nucleobases were found in this meteorite as well, it could be that these molecules are common across the Solar System, as these meteorites and their parent asteroids come from very different places.'
NASA's Lucy mission hopes to visit eight asteroids in the coming decade, including C and D-type asteroids, allowing scientists to discover even more about how these bodies contributed to the formation of the Solar System.
- Rocks and minerals
- Prehistoric
- Read the paper in full published in Nature Communications .
- Find out what else Helena is working on here .
- Follow Charlotte on Twitter .
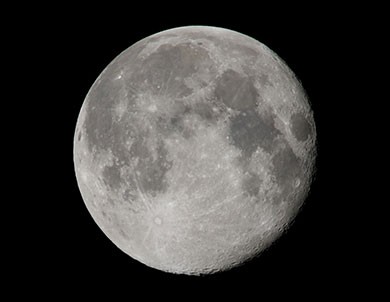
Explore space
Discover more about the natural world beyond Earth's stratosphere.
Related posts
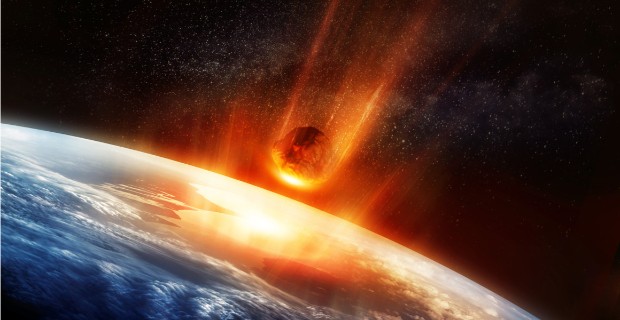
Greenland asteroid struck world recovering from dinosaur extinction
While its impacts are uncertain, it could have caused the world to warm significantly.
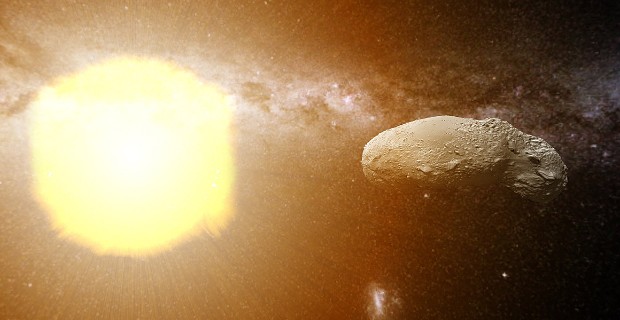
Earth's water may have been formed by solar winds
Solar winds from the Sun could have produced much of Earth's water by irradiating asteroids.
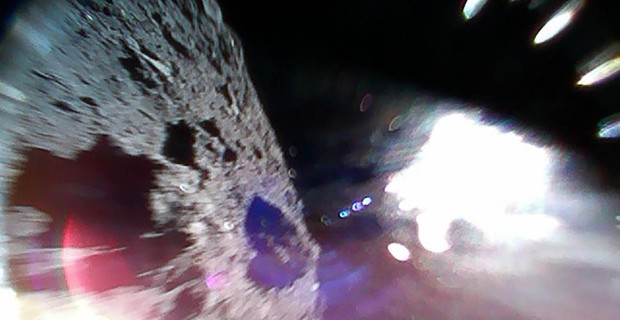
Hayabusa2 lands two rovers on asteroid Ryugu
The rovers have captured the first ever images from the surface of an asteroid.
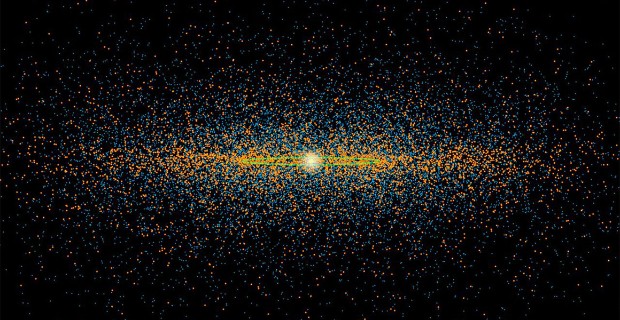
Museum scientist immortalised after asteroid is named in their honour
Orbiting between Mars and Jupiter, the asteroid has been named after the Museum's Head of Collections.
Don't miss a thing
Receive email updates about our news, science, exhibitions, events, products, services and fundraising activities. We may occasionally include third-party content from our corporate partners and other museums. We will not share your personal details with these third parties. You must be over the age of 13. Privacy notice .
Follow us on social media
- Ask An Astrobiologist
- Resources Graphic Histories Coloring Pages Heroes Posters Life in the extremes Digital Backgrounds SciComm Guild

We welcome to SAGANet Dr. George Cooper, Senior Scientist at NASA Ames Research Center, and an expert in meteorites and their potential role in the emergence of life on Earth! You can read more about Dr. Cooper and his work here .
Transcript of this Episode
Greetings, friends of astrobiology. Welcome back to a new episode of Ask an Astrobiologist. This program is the show of the website SAGANet.org, where we celebrate science, diversity in science, and scientists. This program is made possible by contributions from the NASA Astrobiology Program, ELSI, the Earth-Life Science Institute, and the nonprofit Blue Marble Space. My name is Sanjoy Som, but before we start, this is your background quiz, like every month. Mike, if you could put up the background from last month. It is, actually nobody got it right, there was no suggestions for what it is.
Other Recent Episodes
The art of how to become an astrobiologist with dr. aaron gronstal, how & when do molecules become life with dr. henderson "jim" cleaves, from deep-sea treasure diving to exploring the surface of mars with dr. michael meyer.
I was asking you guys to kind of identify what the little mounds were on the bottom left of the image. Those are actually modern stromatolites. Those are microbial mats that grow up towards the sunlight, of course, and they form mounds. They're modern analogs of some of the most ancient fossils of life we know on Earth. The ones from the background from last month are in a biological reserve in Mexico, called Cuatro Cienegas, if I pronounce it right. Some of the oldest ones we have found in the fossil record are over 3.5 billion, and that's with a "b," years old. So really really ancient forms of life that are found today in analog environments.
Speaking of analog environments, what is my background this month? You can see this cold ice desert. It's a location where scientists have gone to test instruments that are destined for Mars exploration. If you know the answer, put it on #AskAstrobio on Twitter or on the SAGANet.org chat, and we'll give you a shout out next month if you get it right. Speaking of very old stuff, this month we're going to talk about even older material, meteorites, and for that we are very lucky to welcome to the program Dr. George Cooper, who is a senior scientist in the exobiology branch at NASA Ames Research Center in California. Welcome, George. How are you?
Oh, fine, Sanjoy, thanks.
Like we like to do in this show, we first rewind the wheels of time a little bit. We are curious about how did young George emerge to become the scientist he is today.
Well, I hadn't really discovered scientists, science until basically college, and just sort of fell in love with chemistry instead of other subjects in undergraduate. From there, one thing led to another.
Why choose meteorites? Chemistry is a very, very broad discipline, right? What got you interested in meteorites and their potential clues to the origin of life?
Well, in graduate school at Arizona State University, my professor, well, my eventual professor, major professor was John Cronin, who maybe some people in the audience have heard of. The reason I ended up with him is that I'd always liked astronomy, having a telescope and things like that. Like I said, in undergraduate I liked chemistry, so it was trying to find an area of study in graduate school that sort of melded those two things together.
He was the perfect person, because meteorites, undoubtedly you cannot talk meteorites without talking astronomy and the solar system. At the same time, the work is basically analytical chemistry. You know, I would say John Cronin, and then there's the Arizona State University meteorite center, I think the world's largest university meteorite center. It sort of came together from there.
That's very exciting. It's clear that you're an astrobiologist. Interests from astronomy and chemistry are merging to guide your field of research today. Let's talk first about meteorites. Could you describe to us what they are, how different they are? You know, there are iron meteorites, there are chondritic meteorites. What are the two differences and where do they form? Why are those important to study in the first place?
Well, the astronomers would take you back to the beginning of the solar system, and now they have photos, actual photos of other forming planetary systems that form out of rock, dust, gas and coalesce into larger bodies, planets, comets, asteroids. The meteorites that I study, when most people say the word meteorite, they're usually thinking of asteroidal meteorites. Asteroids, like comets, are leftover bits of planetoids that didn't form the larger planets like Earth.
Comets, you could have meteorites from comets also, but I think the historical problem is, if you picked one up, you wouldn't know whether it was a comet meteorite or an asteroid meteorite. The meteorites we do have appear to match the spectra of asteroids. Right now, carbonaceous meteorites in particular are pretty much almost all that scientists know of. Probably came from the asteroid belt, from the initial formation of the solar system.
The organic chemistry that's happening in meteorites is not something that happens once the meteorites have arrived on Earth, right? There's organic chemistry that can happen if the meteorites have ice when they are in the faraway solar system?
Right, and even before the bodies of the asteroid, the parent bodies of the meteorite formed, people for example here, but over the years, in Leiden, have shown that on the interstellar grains, the grains that eventually coalesce to become asteroids and comets, even there you have chemistry taking place. You have carbon, oxygen, nitrogen, and a source of energy, photons, radiation. Organics are even made before then. There's a big group here at Ames, Scott Sandford, Lou Allamandola, Michel Nuevo, who do this all the time. They form organics simulating the conditions of interstellar grains, 50 degrees above absolute zero, things like that.
That's probably I would say where the initial organics are formed, eventually to be in the rocks that we have in the lab. After that, the grains, as I say, they coalesce, and there's further chemistry once that water warms up more. If you have chemicals in water, you're going to get more chemistry. So for example, glycine, the smallest amino acid, easily form from formaldehyde, cyanide, and a few other, a couple other things. They're all seen in interstellar space. All the chemicals that amino acids and therefore us, we're made of, are out there.
Once they land on, freeze out on these grains, formaldehyde, cyanide, aldehydes, and ketone, once they form on the grains, as I said before, there's chemistry. When they warm up, incorporate into the larger asteroids as evidence for aqueous alteration, there are two. So two I would say primary sites for organic formation.
We are really a LEGO structure of organic chemistry. If some of those fundamental organic compounds came from meteorites, which are not formed on Earth, but formed in the early solar system, that really means that we are star dust, which is quite humbling, I think, right?
No, that's ...
No, go ahead, sorry.
Yeah, that's the same chemicals astronomers see wherever they look in the universe, which is a good sign for potential life in other places.
Life could have been, if life exists on Mars and elsewhere, of course you have no confirmation of that, but those early, those planets were also subjected to the same early bombardment as Earth, and therefore they also were contributed organic material. In your view it's possible, given the right circumstances, and it's a big "if," that life could have emerged on other bodies, other than Earth, of course.
Oh, yeah, of course. Even Mars last year, I think 2015, maybe it was, the finding of organics on the surface by the Rovers. The paper, I think it was the Journal of Geophysical Research, a big group, said that, well, the organics that we're finding are probably either meteoritic or from some indigenous Mars process. As I say, how the astronomers see these compounds, every place they look, and now obviously land on Earth every day, or we wouldn't have them, the rocks in our labs. But now on Mars, years ago, we saw a comet crashing into Jupiter, so yes, the potential, in addition to the meteorite organics, the further chemistry on Earth, or maybe some also indigenous Earth compounds. Yeah, I think there's a great potential for other life.
All life, potential life in the solar system is shopping for organic matter at the same grocery store, right?
How does one find meteorites on Earth, and how do you differentiate them from other rocks?
Well, there are big groups, for example, that go to the Arctic, and in ice-covered, snow-covered backgrounds, like the one behind you.
I've seen some of the pictures, and they're pretty easy to spot. Most meteorites are darkish and against the snow. You can readily pick them out, but also they're seen to fall a lot of places, and if you're careful and you follow the trajectory, you can find them yourself. For example, the Murchison meteorite, the most famous, was found in Murchison, Australia, in 1969. People saw it fall and saw a big debris field. That's the major type of discoveries. Antarctic, a lucky find. They're transported to museums, most of them, the ones that aren't privately found. Scientists, me and others, can request from museums or Antarctic meteorites again, from, say, the Johnson Space Center, which has a huge collection. Those are the major ways to get them for scientific research.
You have actively worked on the Murchison meteorite itself, which is really cool. Could you tell us a little bit about how you feel when you receive a sample that's as famous as the Murchison, perhaps you can describe to us also why it's famous and what goes through your mind when you hold this rock that 4.6 billion years old?
Well, we had an outreach the other day with students from one of the high schools, somewhat sort of local high schools, and I had powder that is no longer used for research, meteorite powder that we grind up and extract out the organics. But what I did was let them smell it, because if you shake it, the particles are still breaking down to this day, and the original gases from the solar system come out. Most students say it sort of smells like dirt, maybe wet dirt in the country when you walk along the road.
The feeling you mentioned is still something else to think that you're actually looking at, smelling things that are actually closer to 4.6 billion years old. Then it's a process of extracting out organics with normal analytical chemistry means. As far as Murchison, the reason it's I would say more famous is because when it fell in '69, there had been meteorites of course a long time before that, and there had been analysis before that, but mostly they were very contaminated.
Of course, the earlier people had found some indigenous compounds, but a lot were just earthly contaminants. Murchison, as I said a few minutes ago, it was actually seen to fall and picked up very quickly, and on top of that, it had many, many organic compounds. It sort of stood out right away, and the analysis began and people discovered amino acids in it. The older meteorites were thought also to have amino acids, but again, that contamination problem. Murchison sort of settled that question once and for all, and that's why it's the more famous.
You bring up a good point, in the sense that Earth is covered with biology, right? If a rock from space falls on the planet, then the biology that's near it could contaminate it. How are we sure that these organic matter that we find in meteorites are not just Earth contaminating the rock?
Right, right, in almost every meteorite I've looked at, there's Earth contamination, at least some. There's one that's very clean, but most, right, life loves any carbon source. The way people have discriminated against indigenous versus contamination, one of the principal methods was isotopes, and people watching probably have loosely heard of isotopes, different weights of the same compound. For example, carbon, most carbon in nature is 12, weighs 12 on the periodic table. There's a little bit of carbon 13, I think, around 1 percent, I forget the exact number.
With mass spectrometers, very sensitive mass spectrometers, it's quite easy to tell if let's say the carbon in glycine has the Earth ratio of carbon 13 to carbon 12, or if it's higher relatively in carbon 13 than to 12. That tells you right away, that is not from Earth. Geochemists are very good, even with only earthly samples, they can take isotope measurements, let's say of a rock in California or a rock in India, and in some cases tell you what country it's from or what region, rather, it's from, simply by the isotopes and how it was made.
The astronomers see that in space, many compounds are fractionated, that is, these organics I mentioned a while ago, many are higher in the heavier isotopes, of carbon, hydrogen, nitrogen. When they're incorporated again into the parent bodies of the asteroids, fall to Earth as meteorites, that signature, a good deal of it, is still there. That's number one. The second best way is in some cases just as good, is to look for organics that aren't used in biology. That's what people have done a lot since Murchison, look for strange amino acids, because asteroids wasn't a biological process.
It would make glycine with two carbons, all the way up to ... The space process is abiotic, non-biological process, would make from carbon number 2 to carbon 15 for amino acids. It's just thermal dynamics, whereas life on Earth uses very specific amino acids, very specific weights. Life, your body, bacteria doesn't want to make every possible isomer of a compound up to C-10. There's simply, that's a waste of energy. Again, the nonbiological, abiotic process in asteroids, probably comets, make all, including weird ones not used. That's how you tell if it's really from space, is nonbiological compounds.
That's so cool. Meteorites are really the history book of the solar system, and you and your colleagues learn the language of the rocks, really extract the story of what that means for the formation of planets, but also for the origin of life. It's just a fascinating field. Many years ago, I was lucky enough to be in Washington, D.C., and was visiting the meteorite collection you were talking about, and I held in my hand ALH 84001, which is like the meteorite that we know came from Mars. There's not only meteorites that come from far beyond in terms of asteroids that feed the Earth, but also the different planets are exchanging rocks with each other. Have you worked with Martian or even lunar samples?
No, not with the ALH you mentioned, nor moon rocks. My research is primarily the asteroidal meteorites.
Okay, and the fact that rocks can transfer between planets is also I found mesmerizing, because you can tell some stories about the Martian atmosphere from the atmosphere that's trapped in bubbles that form on Mars and that rock was transported to Earth, from which you can analyze using the tools you mentioned. The analytical techniques and the precision you need to make such conclusions is incredible. I was also quite amazed to hear about your colleagues that simulate the solar radiation inside the laboratory to create the conditions that leads to the development of organic matter, that then you can take in the laboratory using your techniques to see what's there. Then does that conform with what we can expect? Wow, it's really cool stuff.
When you were a student, I'm sure like all of us that's been through college, it's not an easy path to navigate, especially when you're after a PhD. I was wondering if you benefited from mentors in that process. Any people that contributed, that helped you out to become the scientist that you are today?
Well, again, graduate school, your best mentor is going to be your professor. Undergraduate, you do have of course professors that some you're closer to than others. Of course, there's always help along the way, but for getting settled in your particular field, for example, the organic analysis of meteorites we're talking about now, the major professor of graduate school is I would say the chief mentor and so I was lucky. I had what I call the professor's professor in John Cronin, who took his work very seriously, and he sat there all day and get everything "i" dotted, everything would be correct when he got up.
I would say that mentor-wise, but you know, at ASU, as far as being in meteorites, you know, you had his postdoc there, Sandra Pizzarello. You had the ASU Meteorite Center, with Carleton Moore and other people there, who this was serious business for them. That always affects a student, as you probably know. It's the whole community of meteorite, in my case the organic analysis of meteorite group.
What did you do after you finished your PhD with an expertise in meteorite studies?
Well, I came to Ames as a postdoc for Sherwood Chang, who's one of the founders of astrobiology. He really worked for years to focus research, get funding for research, and he was the branch chief at that time of the exobiology branch here. He retired years ago. I was his postdoc, so a few years postdoc, and then a position came open, I was lucky to get the research scientist position here. That was pretty much just the trail from graduate school.
Cool, cool, cool. Let's see. The academic environment is one that is changing, and I was wondering if you have any recommendations for minorities who are interested in science and want to become scientists and get PhDs in science.
Well, I guess if you start at undergraduate, the advice, a lot of the advice is the same for everyone. Find opportunities, ask professors, counselors, about internships of things you might be interested in. Let's see. Now there's the Internet, you know, to search. Minority, for example, I knew people at Howard University and Texas Southern. It's always good to try to reach out to people like that who can tell you how they operated around the system, you know, what made life a lot easier. That and I always half-joke, that and get lots of sleep, but health. I would say if you have an idea of what you want, then there's nothing like getting internships, finding the information. Most professors at their schools should have an idea of internships and opportunities. I think that's huge.
Yeah, and students have really nothing to lose for reaching out and contacting an expert. "Hey, I'm really interested in this. What can I do to advance my career?" Most of the time, you'll get a response. All of you who are students who are watching this, don't feel shy about connecting with a scientist.
Most likely they'll be delighted to reply. What do you find most exciting about being an astrobiologist?
Well, again, it goes back to what you mentioned, is looking at these 4.57 billion year old rocks. That is still to me amazing. That is number one, the fact that you can look at something that formed before anyone thought about humans, before there was any thought about, before the Earth actually formed. Now again with the space telescopes, it's just not some delusion. You actually see the same process happening all over the place, over and over again. It really drives home the point that you are looking at formations of planets eventually to be people and bacteria and crocodile. You know, it's just amazing. That I would say is still number one.
Can you paint in your mind and share that with us, perhaps, how you think life arose on our planet? What kind of environment?
Yeah, boy, that's a Nobel Prize question.
Right, that's a tough one.
Maybe in three years, I'll give you an answer. But that's the golden question, you know. We see the compounds, organic compounds in meteorites, but it's obvious that it took from there some more, some additional chemistry on the Earth. For example, right now, we don't see long polymers of proteins, you know, made of amino acids, which is in everything that's life. There's a very small two amino acid peptide, very small piece of a protein, but that's in very trace amounts. It had to take more chemistry from the Earth, and probably additional chemistry in hot regions. People are doing hydrothermal vent simulations, people like George Cody at Carnegie.
Years ago, researchers took amino acids and simply put them on what they simulated to be hot islands, ancient volcanic islands on the ancient Earth, using just heat. You can form linkages, you can join compounds together, which is necessary before you get life. You have to join individual small compounds together, and they could do that with simply heat, by mimicking the many, what had to be the many volcanic hot areas on the ancient Earth. Heat is just the simplest thing to do. I don't know of a complicated scenario for life. People are working on it, but I would start with something like that. We'll, as time go on, ideas will get better and better.
Yeah, it's an active area of research. If I understand you well, the seeds you need to get life started on a planet requires not only solar system chemistry, where you have cosmic radiation that's interacting with ice in the meteorites to create this organic matter, but also the geochemistry, the chemistry that's happening at the interaction of a hot terrestrial or planetary surface, interacting with rocks and water and the chemistry that's happening then, putting those two together, and with the proper conditions, could then arise biology, right? So it's not only a closed planetary ecosystem that leads to life, it's this interaction of the planet with its outer solar system environment.
Exactly. Even though I'm fascinated with meteorite organics, this big Earth wasn't sitting here for nothing billions of years ago. Like I said, undoubtedly there was further chemistry, maybe different chemistry. The organics in meteorites, you can make very fragile compounds that you needed, probably needed for life, for example, pyruvic acid, oxalic acid, things like that, because you're forming them out there, as I said, at 50 Kelvin. Very cold. There are radical compounds that are very stable in interstellar space. When they fall to Earth, they're very reactive. Right away on the Earth's surface, they could have done more chemistry, but again also linking monomers, linking amino acids together, sugar or sugar-derivatives together, to make our larger, the larger polymers we're made of.
Right now, it looks like that had to be an Earth process. Whatever the mechanism, like I said, I sort of favor warm, maybe even hot, at least localized. The ancient Earth, for organics, couldn't have obviously been terribly hot or you wouldn't have had organics, but localized at just the right temperatures. There must have been a very, a huge amount of different temperature regimes, for linking compounds together, I think that would be, the right compounds, and there are many in the meteorites and who knows what were made on Earth. There are the right compounds that if you did have a warm or hot environment, that easily could link, compounds could have been linked together to form larger polymers.
Fascinating. Could you take us to your lab and describe to us what you do in the lab? What kind of instruments do you use to make such detailed measurements?
A few different types of instruments and techniques. The first is really boring analytical chemistry stuff.
Oh, don't say that.
You have to prepare your sample, you have to extract with a solvent, it could be water, it could be a more nonpolar solvent. First you have to extract the compounds out. There are a lot of salts in meteorites. So that's, to keep the story short, you couldn't do the type of analysis I do in the mix of all the salts. For example, in Murchison, and a lot of carbonaceous meteorites, there's lots of sulfate, SO(42-). Murchison, a lot of it is magnesium, calcium.
You have to what we call de-salt. You have to remove those to the side, to get at the organics. Here's where the boring analytical preparation techniques come in. You use resins to de-salt, and you go on and on and it can take forever for one sample. The instrument that finally tells you what the organic is in my case is mostly gas chromatography-mass spectrometry, GCMS. A little bit of liquid chromatography, but that is my, has been my major analytical instrument that actually identifies compounds. I use others for other reasons.
Can you, could you describe what a GCMS is and does in just a few minutes?
Okay, a gas chromatograph, the GC part, you inject, after you've done all the preparation, you inject the sample. The word gas implies, of course, a gas phase. It vaporizes your sample, puts it onto a GC column. A column is a long, glass capillary tube, coated inside with an organic phase. Your compounds, after you inject, land in that long column, and these columns can be 25 meters, 50 meters. Often I've used even longer GC columns. They land in that phase.
Again, back to the gas part, you start heating your gas chromatograph, so your columns inside the gas chromatograph. The organics are now, they've landed on that column. Now you start heating that column, and eventually things boil off. They boil off at different temperatures, because most of the audience knows about a boiling point. Water boiling point is 100 degrees Celsius. The organics on this column, as you slowly heat up your column, they come off at their own particular boiling point in that particular organic phase of the column.
That's the major way to separate organics. They boil off, they're swept out of the column. You're always running a gas through it, an inert nonreactive gas that doesn't react with your compounds, for example, helium or hydrogen. Because each compound has a different boiling point, you could imagine, when these compounds are leaving the column, going to the detector, the mass spectrometer, you'll see each compound come off at a different time. In other words, this gas chromatograph is taking this big goop of a mess of organics in the meteorites, and separating it so you can identify one by one the compounds. You couldn't do it if you simply had all the organics just sitting there in one big mixture.
After they go to the mass spectrometer, the mass spectrometer can tell you the molecular weight of the organics, and it also fragments the compounds. It shoots a beam of electrons at each compound, they break apart, fragment, but they do it in different ways. That's called a fragmentation pattern. The fragments are an ID, fingerprint, of each organic. That's two ways to identify. The retention time, or the time it takes each compound to boil up and come out, and its fragmentation pattern, how it breaks apart. You know that because you run several standards either before or after you've analyzed your sample, and if that standard matches, then you can go back to your sample and say, "Aha, this one had the same retention time and the same fragmentation pattern."
GCMS, the gas chromatography mass spec, is what was used for I think the first big Olympic cheating scandal. I think that was 1990s, maybe Sanjoy, well, you probably weren't born then. I think it was the '90 or '92 Olympics, there was a big doping scandal. And a gas chromatograph mass spec did exactly what I just described. It identified the compounds and so if you were caught, you couldn't say, "No, no, no, the GCMS was wrong," because it's really tough to be wrong when you have a standard and all the properties are identical. It's pretty useful. For example, the water in the background of Sanjoy there, when you melt it, you could analyze it by GCMS to tell what compounds are there. It's a pretty useful technique.
That's so cool. I could talk to you forever about meteorites and organic matter, but I think it's time that we opened the floor up to questions. I'm going to turn to my question screen, which is right here. The first question is from Michael Wong: "What is responsible for choosing the chiral 'handedness' of organic molecules in life?"
That's another golden question. No one knows yet. Scientists know why it works so well. What he's talking about is if, I don't know how diverse the audience is, but if you put up your two hands, you could imagine, pretend, that's an organic compound. You have a left mirror image and a right mirror image. Well, for example, in RNA and DNA, only one of those mirror images of the compound, in this case, let's say ribose in RNA, only one is used in those long strands of RNA and DNA.
Most people from school, high school, have seen the long strands, you have an RNA, a base and phosphate, and it repeats. RNA, ribose, phosphate, base, and it goes on and on and on. But only one of those ribose, only the D mirror image is used. That's what caller Mr. Wong is asking about, how in the world can you get just one? Because if you go in the lab to make, let's say ribose, nonbiologically, like what happened before life, it wasn't a biased process. If you go in the lab now, and do the same thing, you'll get both. You'll get two hands, just like flipping a coin a thousand times. You can't get one coin, only heads.
That is a good question. How did one get picked out? From what we're seeing now with compounds close to sugars, and what my old professor and Sandra Pizzarello saw back years ago, a paper published in Science in 1997, was that some of the amino acids have a slight bias of one of the mirror images. Sometimes the amount, the excess of one of those mirror images vary. It's the L amino acid, which just by coincidence is the one your protein is made of.
Lately we see sugar derivatives, cousins of ribose, that has a lot more of one mirror image, and that's the D mirror image, and maybe it's coincidental now, but the D of the sugars is what life uses mostly. Is that a hint to the answer to that question? What else happened on the ancient Earth? That, what I described came from space, comes from space, what else happened on Earth? People are looking at that. Maybe if this worked, we could do more of it, and see the same thing over and over. I saw that phenomenon since 2001, but we just published it, but I've seen the same phenomenon in these sugar derivatives for over 15 years. People wondering, why the heck aren't you publishing it? You still, I still, you should still look at it in different ways.
The reason it works, I say at first, scientists weren't sure about how it happened, but they know that you can only form, let's say, RNA and DNA if you have one mirror image. That's why it works well. You can't have both, what's called a racemic mixture, you can't have left hand and right hand ribose and go and try to make your DNA. Jerry Joyce at Scripps showed years ago, I think it was '84, a paper in '84, that you don't get very far if you mix both of those mirror images. We know why it works well, but the initial start is still a question.
Cool. Thanks for that, George. Our next question comes from Janet Ashley Pollock. Hello, Janet, thank you for your question. She asks whether comets could also deliver organic material on Earth.
Yes, we just, you know, don't have what we can actually say is a comet, a bonafide comet. Like I say, some asteroids were probably comets. Who knows what, organically wise, what the difference is. We just can't say. There was a paper last year or a few years ago, last year, I think, where they identified glycine on the famous comet now 67P, where the European space craft is landed on. Glycine is the smallest amino acid. They identified small organic molecules, CN, cyanide, a few others, but the larger more, what we think of as biologically relevant, I don't think is known about comets yet.
Even if there were only those small molecules, formaldehyde is one of them. There's formaldehyde is just CH20. HCN, cyanide, another compound. Even if this only, they are the only ones on comets, if they landed on ancient Earth, the chemistry you could get, the further chemistry, the building of larger compounds from that, I think would be pretty significant. Again, if you have formaldehyde, CN, which are in comets, right away, not right away, but pretty much, you're going to get glycine and other amino acids. Just the small molecules would have been I think huge, as far as further chemistry from comets.
Thank you. The next question is from Gina Riggio. Hi, Gina, thanks for your question. She asks, "What is an example of an unexpected organic compound that you have recovered from a meteorite?"
Oh, the most unexpected. I would say it was an organic phosphorous compound that was fairly unusual bond. For example, DNA and RNA are organic phosphorous, but that's not, as far as we know, not in meteorites. That's phosphorous with four oxygen around it. The RNA, each phosphate is bonded to a ribose, and then another phosphate and then another ribose and on and on and on. That's PO4, phosphorous with four oxygen. The most surprising that I can remember is, the organic phosphorous we saw years ago bonded directly to a carbon, and it has different organic groups attached to the carbon. They're called phosphonates, not phosphate, but phosphonates. I think that, compound-wise, not phenomenon-wise, but compound-wise, is probably the more surprising.
Cool. Janet asked a question again, about whether you have any, she's Canadian, and she's asking if you have any Canadian colleagues who do some of the early life, early Earth type studies.
Not myself, I do not, but there are people in Canada who like meteorites also and analyze organics. But not personally, no.
Janet, you should check out the Center for Planetary Science and Exploration, which is in London, London, Ontario, I think? They might have, it's at Western, they might have a department there that you might find some collaborators. The next question is by Abhishek Soni. He asks, "What can an environmentalist do with astrobiology?" If you're a modern ecologist, environmentalist, how can that give you insights into astrobiology? I think that's where that question is going.
Well, I haven't thought of the environmental connection before. As far as astrobiology, you said? Between the environment and astrobiology?
Can you, yeah. No, I can't think of a, when I think of environmentalism, I'm thinking of trying to save the planet from its current, you know, head towards destruction, climate change and the rest. I'm sure there must be some connection. The astrobiologist that I spoke of before, they go all around the Earth searching for meteorites. Obviously the ice melting where they go collect meteorites, so I'm sure they would have a really strong bent on that particular question.
It's true, and analog studies, Abhishek, are a modern way of studying astrobiology. Look at analog sites that are similar to some conditions you're exploring in other worlds, and if you have some expertise in the ecology or the local biogeology that's going in there, then you can make some educated conclusions about the plausibility of that environment to be similar to one elsewhere.
There are so many questions that are pouring in right now. Thank you all for all these great questions. We're probably not going to have time to get through all of them, but we'll do the best we can. What is the next question here? Oh, it's a question by Jacob Haqq-Misra. Hello, Jacob, thank you for your questions. He asks, "What are the main geochemical differences between meteorites that originate from Earth compared to from Mars?"
You mean the meteorites that fall to Earth?
Martian meteorites that I know of, again, I haven't studied, but I think the majority are more rock-like. One or two have, they have found traces of organic compounds, but they look like they come from a heating process, an igneous process. Certainly the big complex mixture or organics that we see in asteroidal meteorites that fall to Earth, cannot be seen in Martian meteorites. I would say they're more rock-like, more planetary-like, less organics.
Yes, meteorites from Mars are essentially like lava flows that then cooled off and became rock that were then ejected by some sort of impact and landed on Earth. Whereas meteorites are more primitive silicon material that are what the planets are made of in the first place. I think that's what you're after, Jacob.
Yeah, more pristine.
More pristine, that's it. The next question is by Graham Lau. Hello, Graham, thank you for your question. "What do you think the odds are that we have Venusian meteorites already in our collections, but just don't know about them?"
Oh, well, the ones in the collections, they're pretty well characterized. Every now and then I will hear something about a meteorite, possible rock from Venus, but I don't know the people who think, the geochemists, the geologists actually, who think about that type of thing, I don't know what the latest count is, but I do hear about that now and then. I just couldn't give a good answer.
It's a good question, Graham. I don't know either. Venus meteorites, that would be cool.
There's probably some rocks from a lot of different places on Earth, we just wouldn't recognize it, if we stepped on it.
Yeah, because you have to find them in specific conditions to recognize them as meteorites, it's not always obvious. The next question is Gina again, she ask a very good one, in terms of, you were talking about the column in your lab that's like 25, 50 feet, or meters, or even longer. So she asks, "How does that fit in your lab in the first place? How many compounds can you separate using that technique?"
Well, I plan to ask the Center management for a nice long lab. No, the columns we're talking, the ID is a millimeter less, the internal diameter, so they're tightly coiled. They're made of flexible polyimide material. You can imagine, take 50 meters and coil it up into a circle of about, let's say a foot across. That fits in your gas chromatograph oven.
Gotcha. How many compounds can you separate? Like a hundred in the sample?
Oh, more than hundreds, more than hundreds. Yeah, in a single run, you could have, you know, you can't even count the number in the meteorite, it's just a strange number.
Sukanya Singh asks if you've ever detected a compound that is not organic and that you're like, whoa, that's a really weird one. That should not belong here. Or is everything that you've discovered kind of expected from the cosmic chemistry?
I do see nonorganic compounds now and then, as just simply you don't get all of the inorganic compounds out. For example, sulfate, SO(42-) I mentioned. You try to get out most, as I said, but you see sulfate, phosphate, traces of it, sometimes in your analysis. Sulfite, SO3, and there's probably one or two others. Those are the principal nonorganics that I'll see now and then.
Cool. Kai Pisarek asks about the enantiomeric excess, so the excess of one chiral molecule versus another. What is the excess, I guess in meteorites, and couldn't you use that excess to say something about biology? How big does the excess need to be in order to make conclusions about biology?
Okay, well, going back again to before I started, and I mentioned the '97 paper, Cronin and Pizzarello. Before that, there was a paper looking at biological amino acids. Ingall and Mackell, and I forget the year, around 1990, of alanine, a biological amino acid. Again, people are always leery of biological, if you say there's an enantiomeric excess, but it was good work. We'll have to see about more about the biological amino acids. When you get to the '97 paper, Cronin and Pizzarello, as I said, some of the strange amino acids that are not in biology, that's there's no question that they came from space, so if you see an enantiomeric excess, you can say that's indigenous for a fact.
Those enantiomeric excesses, or in other words, one hand more than the other, the excess of one mirror image of the amino acids, back then they were in the range anywhere from 1 percent, I think, up to 10 percent. This was more than one amino acid. They concentrated again on the nonbiological and nonprotein amino acids. That's another thing. You want ones that aren't laying around everywhere in proteins. They were in the range of 1 to 10 percent. Other people have done, looked at those compounds, too. The paper, boy, it's early morning, I forget the year of the paper, but Glavin and Dworkin, I think Jamie Elsila, too, they did a big survey of meteorites and they found large excesses in some of the same amino acids. I think they went up to 20 percent. So 20 percent excess of one enantiomeric, one mirror image.
Then Pizzarello, Sandra Pizzarello found 50 or 60 percent excess, you know, one over the other, in other larger amino acids. I think these were isoleucine derivatives. Mostly with the amino acids, though, you're talking about that range of 5 to 10 percent excess, just generally. The ones we saw last, now switch to sugar derivatives, away from amino acids, so last year in June, we published that. We went compound by compound, increasing carbon number, so we went from glyceric acid, so instead of the sugar, glyceraldehyde, if you have your organic chemistry book, the oxidized form is glyceric acid. You add an oxygen, and you get an acid from that sugar. We started with glyceric acid, which is three carbons.
Then we went to four carbons, to five carbons. Five carbons is where you get to the relative of ribose, the very close structural analog of ribose. Then we went to six carbons, where instead of glucose, you have gluconic acid. Most of those six carbons are rare on Earth, gluconic is not. Back to the question. At the first carbon number, there were glyceric, it was fifty-fifty. No matter what meteorite you looked at, fifty-fifty. Now if you got a very contaminated meteorite, you knew it was contaminated, you can see a lot more D-glyceric. But for normal, mildly clean or super-clean meteorites, the three carbon was fifty-fifty.
As we went up in carbon number, that spread increased. We went to C-4, it was three to one D [inaudible] and threonic. We went to the five carbon, the ribonic acid, the cousin of ribose, that was eight or nine to one D. You went up to the relatives of glucose, and it was only D. We didn't see any of the other mirror image, the L. I would still not believe it, except the weird non-earthly ones are the same way.
There's no strict boundary, right? There's no strict definition that above 50, it's biological, and below that it's not. It really depends on what compounds you're talking about and so on, right?
Oh, right, right. We're talking about on a molecular basis, what's found in life and therefore can contaminate your experiment, and what's not found in life. They all show this increase in excess of one mirror image. I should modify one ...
We're running close on time.
Oh, okay, okay.
I just want to give the opportunity of having one last question before we end. That is, Damian Sternmann, hi Damian, thanks for your question. He is asking perhaps a more speculative question, because life on Earth and life on other terrestrial planets probably came from the same building blocks. He asks, "Do you think, and speculatively, would alien organisms also use peptides and DNA and similar stuff that we're made of?"
That's another good question, but I've heard astronomers say, we see the same stuff everywhere. They look in space. I think there's a very good chance, yes. They might use a different ...
Figure this one out, Damian, and then let us know. That's Nobel Prize type results.
Yeah, they might use a different mirror image, but same compounds.
George, I know you're a very busy person, and I'm so grateful and thankful that you took time to talk to us today on SAGANet. It's been wonderful having you. Best of luck on your research, and we hope to talk to you again in the future. Those of you who are watching, thank you for your questions. #AskAstrobio. Don't forget to let me know if you know what's behind me, where is that picture taken from. Until then, we'll talk to you all next month, for a new episode of Ask an Astrobiologist. Please stay curious. Bye-bye.
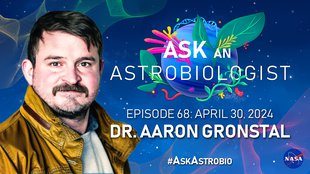

An official website of the United States government
The .gov means it’s official. Federal government websites often end in .gov or .mil. Before sharing sensitive information, make sure you’re on a federal government site.
The site is secure. The https:// ensures that you are connecting to the official website and that any information you provide is encrypted and transmitted securely.
- Publications
- Account settings
Preview improvements coming to the PMC website in October 2024. Learn More or Try it out now .
- Advanced Search
- Journal List
- Cold Spring Harb Perspect Biol
- v.2(3); 2010 Mar
The Organic Composition of Carbonaceous Meteorites: The Evolutionary Story Ahead of Biochemistry
Sandra pizzarello.
1 Department of Chemistry and Biochemistry, Arizona State University, Tempe, Arizona 85287-1604
Everett Shock
2 School of Earth and Space Exploration, Arizona State University, Tempe, Arizona 85287-1404
Carbon-containing meteorites provide a natural sample of the extraterrestrial organic chemistry that occurred in the solar system ahead of life's origin on the Earth. Analyses of 40 years have shown the organic content of these meteorites to be materials as diverse as kerogen-like macromolecules and simpler soluble compounds such as amino acids and polyols. Many meteoritic molecules have identical counterpart in the biosphere and, in a primitive group of meteorites, represent the majority of their carbon. Most of the compounds in meteorites have isotopic compositions that date their formation to presolar environments and reveal a long and active cosmochemical evolution of the biogenic elements. Whether this evolution resumed on the Earth to foster biogenesis after exogenous delivery of meteoritic and cometary materials is not known, yet, the selective abundance of biomolecule precursors evident in some cosmic environments and the unique L-asymmetry of some meteoritic amino acids are suggestive of their possible contribution to terrestrial molecular evolution.
Meteorites undoubtedly showered the early Earth with various organic molecules, including amino acids with excesses of the l -form. Their delivery might have aided molecular evolution and the choice of the enantiomers found in living systems.
INTRODUCTION
Why meteorites are part of the discourse about the origin of life.
The studies of meteorites have long been part of investigations and discussions about the origin of life for the reason that some of these extraterrestrial bodies have reached the Earth containing abundant carbon since its accretion, provide a natural sample of abiotic organic chemistry, and may offer insights on the possible environments and physico-chemical processes that fostered biogenesis. These conditions are entirely unknown because geological and biological processes of over four billion years have long eradicated any traces of early Earth's chemistry. On the other hand, we know that life has embarked in a long evolutionary path all through its recorded history and it seems reasonable to extend to its unknown beginning the same evolutionary nature. Albeit a posteriori and without knowledge of the actual chemical steps that carried this evolution, therefore, the single assessment one can safely make about life's origin on the Earth is that it must have been an emergent process, through which biogenic atoms and molecules gained the complex associative and interactive states we observe in even the simplest forms of extant life. It is then easy to see why the discourse about the origins of life has been multidisciplinary, broad based, and fostered many theories, all of which, with the notable exception of the panspermia hypothesis (e.g., Crick and Orgel 1973 ), accept the fundamental emergent nature of life from simple molecules.
In exobiological (as well as astrobiological) terms, it has been proposed that life's fundamental evolutionary nature might have extended beyond its origin and might be rooted in the abiotic cosmochemical evolution of the biogenic elements. C, H, N, O, P, and S are known to be present as diverse and often complex organic molecules in a variety of extraterrestrial environments (Lazcano 2010) and their long cosmic history has supported the idea of a possible exobiology. However, its analytical basis comes from the study of carbon-containing meteorites that have provided the only natural sample of chemical evolution large enough for direct laboratory analyses. Uniquely, therefore, carbon containing meteorites record for us the abiotic organic chemistry that preceded life's origin and may as yet reveal whether it is realistic to assume that these or similar materials, i.e., either by direct delivery or analogy of formation, might have fostered or even inducted molecular evolution toward biogenesis.
The Early Solar System, Meteorites, and the Possible Survival of Cosmochemical Evolution
The meteorites that reach the Earth are for the most part fragments of asteroids, i.e., of those small planetesimals that orbit the Sun in great number between Mars and Jupiter. By the Titius-Bode law of a regular spacing of planets from the Sun, their orbit should be occupied by a planet; it is believed, however, that the small chunks of early solar materials reaching this area fell under the strong gravity of the already formed giant planets and were either scattered throughout or left unable to coalesce. That is how we still find them today, joined by icy objects from more distant locations of the solar system that were brought in by further dynamical evolution of giant planets' orbits ( Levison et al. 2009 ). With their crowding, hazardous orbits, and constant collisions, all of these bodies put fragments on route to the Earth and have done so through the ages. The importance of meteorites for the study of prebiotic chemistry is a result of this failed planet formation and not just for their obvious delivery but also because many of the asteroid belt objects never had their composition drastically transformed by gravitational high temperatures and pressures as larger bodies did. Their meteoritic fragments, therefore, may carry unaltered a pristine record of early solar system chemistry as well as allow the deciphering of its cosmic history.
The meteorites that best fit this description are the carbonaceous chondrites (CCs), a primitive subgroup of stony meteorites having an elemental composition that is very similar to that of the Sun and the universe overall. As their name indicates, CCs have the distinction of containing several percent amounts (∼1.5%–4%) of carbon, which is for the most part present as organic materials. These meteorites are aggregate rocks, i.e., consist mainly of a matrix made of packed together hydrous and anhydrous silicates that do not show signs of metamorphism or alteration by high heat. However, as part of their small planet parent bodies, CC mineralogy also shows that these rocks had experienced a liquid water phase as well as the effects of impact shocks. For example, a recent measurement of the optical activity of three CC surfaces (Arteaga et al. 2010) showed a circular birefringence bias to negative values that the authors attribute to chiral fractures and distortions in the clays following mechanical forces. The meteorites' aggregation also captured various inclusions; the chondrules, to whose name CCs owe their classification, are round beads of glassy appearance that have re-crystallized from a melt, i.e., high heat, and bring witnesses to the variety of materials and processes that must have contributed to CC parent bodies' formation ( Fig. 1 ).

A CR2 meteorite stone found in the Antarctica Graves mountains (GRA 95229). The open faces show the large chondrules that characterize this family of meteorites. Chondrule and other inclusion abundance reduce the amount of matrix where organics are found to about 30% of the geology.
Overall, these meteorites do not seem to differ much from terrestrial rocks, a similarity that has not helped their collection or preservation because, if not seen to fall and promptly collected, they easily disappear in the environment. The Murchison meteorite was exceptional in this respect because it fell at the very eve of lunar samples' return in 1969 and was analyzed directly by NASA laboratories as a possible analog of those samples. One hundred kilograms of this meteorite were recovered and have been used in 40 years of analyses for probably the most comprehensive study of any extraterrestrial organic material to date. As a result of this focus, the Murchison meteorite composition has long been considered representative not only of meteorites of the same type ( Pizzarello et al. 2006 ) but, often (e.g., Luisi 2007 ), also of the capabilities of abiotic organic syntheses in general. Given our yet tentative knowledge of cosmochemical environments, it is not surprising that the latter assumption turned out to be premature and a new group of pristine meteorites found in the ice fields of Antarctica, the Renazzo-family of chondrites or CR, have been offering a novel view of the possible synthetic outcomes of abiotic chemical evolution as well as of its prebiotic relevance.
The Abiotic Organic Composition of Meteorites: Prebiotic Traits and Biochemical Counterparts
In spite of exhaustive chemical analyses, we still have a very vague idea of where Murchison organic materials are actually located vis-a-vis the inorganic components in the meteorite. The only successful description so far was obtained by X-ray microscopy of the meteorite surfaces after their exposure to selective staining with OsO 4 vapors ( Pearson et al. 2007 ). From these analyses, they appear to be broadly distributed within the matrix, intermixed with hydrous silicate components. As in other CCs, Murchison organic materials can be broadly described in terms of their solubility in aqueous and organic solvent systems, a practical characterization that nevertheless leaves room for missed analytical targets and the possibility of unknowns (e.g., Deamer 1985 ). Insoluble and soluble components represent respectively 70% and 30% of total carbon and, within their molecular range, are both very complex and fundamentally heterogeneous.
Murchison Insoluble Organic Material (IOM)
The larger portion of Murchison organic carbon is often referred to as kerogen-like because, like terrestrial kerogens, it is an insoluble macromolecular material of complex composition that is not known in much molecular detail; its average elemental abundances are C 100 H 46 N 10 O 15 S 4.5 . The bulk of the IOM can be inferred only indirectly from spectroscopy (e.g., nuclear magnetic resonance and infrared) and by decomposition studies, where it is pyrolyzed by heat or oxidized into its fragments. These analyses suggest a general structure composed of aromatic ring clusters, bridged by aliphatic chains containing S, N, and O, with peripheral branching and functional groups. By transmission electron microscopy, most of the IOM appears dispersed and amorphous but ∼10% of it is found as self-contained nanostructures ( Fig. 2 ), spheres as well as tubes, of diverse elemental composition that varies from close to pure graphitic C (> 99%) to containing several percent amounts of O, N, and S. The IOM also contain minute amounts of “exotic” carbon, so called because it was likely formed in the envelopes of stars prior to the formation of the solar system.

Scanning electron microscope image of the GRA 95229 acid residue showing an abundance of submicron-sized spherical carbonaceous particles. The particles are solid, single, and agglomerated with the largest close to 500 nm in diameter. The residue is deposited onto a carbon planchette and imaged with 5 kV electrons and current of 98 pA (reprinted with express permission from Laurence Garvie).
On the whole, the large compositional heterogeneity of the IOM as well as the diversity of its phases strongly suggest that this material is the complex end product of cosmochemical regimes and environments that varied greatly. On the other hand, in spite of being insoluble in acids and solvents, the IOM can free several individual compounds under conditions of high temperature and pressure similar to those of terrestrial hydrothermal vents (300°C, 100 MPa) ( Yabuta et al. 2007 ). These are mainly a variety of aromatic and heteroaromatic hydrocarbons but also smaller noncondensed molecular species and a suite of alkyl dicarboxylic acids up to C 18 chain length. In addition, the hydrothermolysis changed the IOM's chiral response to the Soai autocatalytic reaction in that it displayed a statistical R-chiral bias prior to the treatment but not afterwards ( Kawasaki et al. 2006 ), suggesting that some chiral species are present in Murchison IOM but cannot be detected at the molecular level. These experiments show that portions of the IOM macromolecular structure can be modified at the molecular level, exchange species with the soluble organic pool, and possibly represent materials caught in flux between aggregation states. We may also assume that, were meteoritic materials exposed to hydrothermal conditions or prolonged exposure to water upon their fall, IOM release might have made an important contribution to the organic pool of the early Earth when CCs delivered an estimated 1%–3% of their weight in carbon during the early impact period ( Mautner et al. 1995 ).
Murchison Soluble Organic Compounds
The soluble organic compounds of the Murchison meteorite make up an abundant and diverse group of well over a thousand molecular species that vary from smaller water-soluble compounds such as amino acids and polyols up to 30-carbon‐long nonpolar hydrocarbons extracted only with solvents ( Table 1 ). As their large number indicates, they are also present in multiple isomeric forms up to the limit of their solubility. This diversity is observed throughout most of the various compound types and is often a sign of their indigeneity because it contrasts starkly with the structural and functional selectivity displayed in biochemistry. It has been analyzed in particular detail for Murchison amino acids. For example, all the possible α-amino alkylamino acids up to seven-carbon were identified in Murchison extracts based on the reference of synthesized standards and several eight- and nine-carbon homologous species could also be easily recognized by chromatography-mass spectroscopy on the basis of their spectra even if their standards were not available. Similar large abundances of N-substituted, cyclic, β-, γ-, δ-, and ε-amino acids were also found and the total number of meteoritic amino acids can be placed at over one hundred. In contrast, the whole of terrestrial protein is made up of just 20 amino acids.
Classes of organic compounds in the Murchison meteorite.
Within this overall diversity, several components of Murchison's organic suite have identical counterparts in the biosphere. Eight of the meteorite amino acids are also found in proteins (glycine, alanine, proline, valine, leucine, isoleucine, aspartic acid, and glutamic acid) and numerous other compounds are encountered in terrestrial metabolisms, as shown in Table 1 . A very interesting similarity with biochemical traits was found in a group of chiral amino acids not present in terrestrial proteins, the 2-methyl 2-amino acids, which display in Murchison l -enantiomeric excesses ( ee ) that, if not as large, have the same configuration ( l -) of terrestrial protein. The ee were first discovered in the diastereomers of the seven-carbon 2-amino 2,3-dimethylbutaoic acid ( Fig. 3 ) ( Cronin and Pizzarello 1997 ) and were later established for the whole homologous series of these chiral compounds up to eight-carbon long; their magnitude varies within the meteorite and is largest, up to 18%, for isovaline (2-methyl-2-aminobutyric acid). As the biochemical structures and functions of all life today are dependent upon the exclusive chiral homogeneity of their polymers, it appears reasonable to assume that a homochirality, albeit of unknown origin, was also essential to the origin and/or evolution of life. The ee of meteorites represent the only case so far of molecular asymmetry ever measured outside the biosphere and their indigeneity is supported by compound specific carbon-, and hydrogen isotopic data obtained for d -, and l -isovaline enantiomers ( Pizzarello et al. 2003 ; Pizzarello and Huang 2005 ).

Chemical structure and chromatographic elution of the Murchison 2-amino 2,3-dimethylbutyric acid diastereomers.
Overall, the study of Murchison has disclosed detailed insights on the capabilities and possible range of abiotic syntheses in cosmochemical environments. We have learned that this abiotic chemistry can form organic materials of considerable complexity and include compounds similar or identical to biomolecules. Particularly captivating is the finding of chiral asymmetry in abiotic amino acids and, although less defined at the molecular level, the fact that some macromolecular and inorganic phases of the meteorite show signs of optical activity is intriguing as well. Considered as a whole, these data support the conclusion that molecular chiral asymmetry preceded biochemistry.
Nevertheless, these studies also leave many questions unanswered as to the prebiotic potential of an organic suite of Murchison-like composition. In fact, the large heterogeneity of Murchison organic inventories and the apparent randomness involved in their formation led to question the means and opportunities by which such a diverse mixture of molecules, a majority of which are thermodynamically stable end products (e.g., carboxylic acids and hydrocarbons), could find an evolutionary path toward the selectivity and functional specificity displayed by even the simplest biochemistry.
RECENT RESULTS
The cr antarctica finds.
Recorded falls of carbonaceous chondrites have been few (37 to date, since the first registered in 1806) and this record is needed, because these meteorites resemble terrestrial rocks, are porous in nature, and quickly disappear into the environments if not spotted soon. For the same reason, their organic analyses have also become increasingly limited in scope with their years of terrestrial residence, due to the ease with which CCs acquire biochemical contaminants. Fortunately, several of the meteorites recovered in Antarctica are found unspoiled because of the unique shelter of the glaciers, where falling meteorites are quickly covered by snow, remain buried within the ice, and resurface only when the ice sheets, flowing toward the sea, encounter the obstacle of a mountain.
Renazzo family of meteorites make up a recent classification (CR) of several Antarctic “finds” that have petrology closely similar to that of the Renazzo meteorite, a CC that fell in 1864 and long remained unclassified. Two CR2 1 meteorites (the GRA95229 and LAP02342 2 ) were analyzed recently for the major groups of organic compounds known to be present in the Murchison meteorite ( Pizzarello et al. 2008 ; Pizzarello and Holmes 2009 ) and have shown an organic composition that differs dramatically from that of Murchison and, in fact, from any seen before in carbonaceous meteorites ( Fig. 4 ). Their organic suite is composed mainly of water-soluble compounds, between which N-containing amino acids and amines are predominant. Ammonia is the single largest component of the suite, whereas hydrocarbons and carboxylic acids are only minor components. Novel were also the abundant distributions found within CR2 amino acids, where the shorter chain-length molecules of a homologous series, e.g., glycine, alanine, and α-amino isobutyric acid, are overabundant compared to longer chain species and, in effect, account for most of these compounds' abundance. Several reactive compounds are found in these meteorites as well, such as aldehydes, tertiary amines, and the hydroxy amino acids serine, threonine, allo threonine, and tyrosine ( Pizzarello and Holmes 2009 ); the latter two groups of compounds were never detected in Murchison.

Comparative plot of major soluble organic compound abundances in the Murchison and CR2 meteorites (GRA 95229 and LAP 02342 shown).
Another difference between CR2 and CM meteorites is found in their respective content of enantiomerically enriched chiral molecules. In CR2s, the same amino acid species having ee in CMs display this trait to less extent (GRA95229) or not at all (LAP02342), whereas the abundance distribution of some of the meteorites' diastereomer amino acids allowed the inference of an original asymmetry of their precursor aldehydes ( Pizzarello et al. 2008 ). This somewhat indirect reasoning concerns the diastereomers of the amino acid isoleucine and can be explained as follows. The molecule [2-amino3-methylpentanoic acid, CH 3 CH 2 -C*H(CH 3 )-C*H(NH 2 )-COOH] contains two chiral centers (C*) and can be present as two different compounds (depending on the possible distribution of the methyl branching along the alkyl chain), each with two enantiomers, i.e., the pairs of d -, l -isoleucine (ile) and d -, l - allo isoleucine ( allo ) (called diastereomers and shown schematically in Fig. 5 B). Only l -ile is present in terrestrial proteins, whereas all four diastereomers are found in meteorites.

Possible formative pathway of the isoleucine (ile)- allo isoleucine ( allo ) diastereomers in meteorites. ( A ) The cyanohydrine reaction. ( B ) Schematic of the distribution of ile and allo following the same reaction with a 2-methylbutyraldehyde precursor. ( C ) A chromatogram of the ile- allo diatereomers in the GRA 95229 meteorite.
A possible reaction for the formation of amino acids in meteorites is the addition of HCN to ketones and aldehydes in the presence of water and ammonia ( Fig. 5 A) (e.g., Peltzer and Bada 1978 ). Although producing an asymmetric carbon in most cases (and therefore a chiral molecule), this type of synthesis is nonstereospecific because the HCN addition would be random and give equal amounts of d - and l -enantiomers. However, the reaction results become more complex for longer aldehydes that already contain an asymmetric carbon, for example, in the synthesis of the four ile and allo diastereomers from dl 2-methyl butanal. In this case, were an ee present in the aldehyde, e.g., of the (S) configuration, those amino acids that carried the S-portion of the molecule through their synthesis (shown between dotted squares in Fig. 2 B) will be more abundant than their respective enantiomers. In the above example, this would be the (RS) allo and (SS) ile compounds or, in the formalism used for amino acids, d - allo and L-ile. Such was the distribution of isoleucine diastereomers found in the CR2 extracts ( Fig. 5 C) that, on the basis of the above formative premise, was interpreted to signify that their precursor aldehyde enantiomers carried an original S-bias to the meteorite's parent body.
Overall, the compositional differences between CR2- and Murchison-type meteorites make stark contrasts. Where the heterogeneity of Murchison compounds easily points to the difficulties that a primordial “soup” would encounter in molecular evolution, CR2 organic distributions and abundances have the unquestionable prebiotic appeal of being over abundantly water-soluble, N-containing, and of low molecular weight. Regardless of how CR2 organic material came to be, it is also clear that an unknown combination of elemental composition, energetic availabilities, and cosmic contingencies made CR2 precursor environments capable of a de facto selectivity of such “prebiotically desirable” molecular species.
Whether CR2 meteorite parent bodies would fit the new category ( Levison et al. 2009 ) of “trans-Neptunian” objects or not, certainly the formative environments and histories of their organic materials must have differed from those of CMs. That the known ee -carrying amino acids as well as their ee are in lower abundance in CR2 than CM meteorites, whereas ee appear larger in a precursor aldehydes, would seem to further allow the general inference that abiotic organic pools in chemical evolution were diverse and differed in both their composition and exposure to asymmetric effects.
The Long Cosmic History of Meteorites' Organic Materials
The formation of meteoritic organic compounds was actively debated after Murchison's fall and the revelation that a large variety of extraterrestrial organic molecules with counterparts in the biosphere could be made abiotically. Clearly, to know the syntheses and locals responsible for their formation may have profound significance for the origin of extant life and even a broader exobiology. The earlier hypotheses all focused on solar system processes and, of these, the more influential were the suggestion of possible Miller-Urey type ( Miller et al. 1976 ) syntheses in small planets, following production and recombination of radicals, and of catalytic, FisherTropsch-type, processes in the early stages of the solar nebula, where carbon monoxide could have undergone hydrogenation to hydrocarbons and other compounds (Lancet and Anders 1970). Eventually, the history of the organic compounds in carbonaceous meteorites was elucidated, at least in general terms, by the stable isotope analyses of several compounds and compound classes in the Murchison meteorite.
The isotopic composition is a good indicator of any molecule's synthetic history because the mass differences between isotopomers result in energy differences in their bond formation and may lead to a mass dependent fractionation, which becomes diagnostic of the physico-chemical conditions affecting those reactions. Ultimately, isotopic fractionation is a function of zero point energy difference between isotopomers (ΔE) and local temperature, in the form: exp(-ΔE/T). In other words, the larger the energy difference between isotope bonds and the lower the temperature, the greater the potential for heavy isotope enrichment. Between the biogenic elements, therefore, hydrogen has the potential for the most enrichment in 2 H (deuterium, D) at low T, because of the high relative mass difference of D/H isotope pair (2/1 u, e.g., compared to 13 C/ 12 C = 1.084). The most dramatic demonstration of these capabilities is given by the spectroscopic observations of the D/H ratios of molecules formed in the dense clouds of the interstellar medium where temperatures are in the 10–30K range. Over a hundred such molecules have been described (e.g., Roueff and Gerin 2002), many of which show extremely high D/H ratios. For example, the average D/H ratio in terrestrial organic compounds is approximately 1.5 × 10 −4 , whereas a D/H ratio as high as 0.33 has been observed in the interstellar molecule D 2 CO/DHCO ( Loinard et al. 2000 ).
Most Murchison compounds were found enriched in D and 13 C to varying degrees and these data, which alone suggest a relation between such molecules or their direct precursors and cold synthetic environments, led to a general theory of formation of meteorite organics that involved interstellar as well as parent body processes. By this hypothesis, icy asteroidal bodies accreted with abundant volatiles, including water and deuterium-rich interstellar organics that, upon warming and a subsequent period of aqueous phase chemistry, yielded the various soluble organic compounds of meteorites.
The possibility of parent body aqueous syntheses seems confirmed by the likelihood that at least some of Murchison amino acids were formed via a Strecker-like reaction of precursor aldehydes and ketones, ammonia and HCN ( Fig. 5 A). The evidence supporting this hypothesis is the finding in the Murchison meteorite of comparable suites of α-amino and α-hydroxy linear acids (although this correspondence is not valid for the α-methyl compounds) and of imino acids (e.g., Pizzarello and Cooper 2001 ). These are compounds in which two carboxyl-containing alkyl chains are bonded at the same amino group and would likely result from a Strecker synthesis, e.g., when an amino acid product becomes the reactant in place of ammonia ( Fig. 5 ).
However, there are isotopic as well as molecular trends within the Murchison organic suite that reveal significant formative distinctions between individual compounds and cannot be accounted for by any simplified model. For example, not all of Murchison amino acids fall in the same range of deuterium enrichment, and asymmetry-carrying 2-methyl amino acids display far larger δD values than the 2-H isomers ( Fig. 6 ). Because a similar branched versus linear difference in D-enrichment was also observed between 3- and 4-amino isomers, it seems reasonable to assume that branched molecular species were processed in cold environments to a different degree than the linear ones. On the other hand, 2-, 3- and 4-amino acids also show different trends of 13 C abundance with increasing chain lengths, which decreases in the case of the 2-amino acids while remains level, or even slightly increases, in the case of the others. That is, within each level of D-enrichment, various processes of chain elongation seem to have been possible. The obvious conclusion from these Murchison detailed analyses is that diverse cosmic regimes and synthetic processes might have participated in producing the organic composition of this type of meteorites.

The hydrogen ( A ) and carbon ( B ) isotopic distributions of Murchison and CR2 amino acids.
The isotopic analyses of CR meteorites added to the above scenario. The δD differences between 2-amino acid types are still present and further magnified, with the two GRA95229 2-methyl amino acids analyzed showing the highest δD values (+7200‰) ever measured for an extraterrestrial molecule by direct analyses. However, δ 15 N values determined for CR2 amino acids have a distribution between molecular subgroups that is opposite to the one of their δD values, with 2-H amino acids having higher δ 15 N than 2-methyl amino acids (Pizzarello and Holmes 2008).
Because of the near absence of molecular 15 N values for cosmic environments 3 , only theoretical considerations can be offered for the CR2 findings. The ones offered by Charnley and Rodgers (2002 , 2004, 2008) describe a mechanism for higher nitrogen fractionations in regions of the ISM, where the enhanced density and pressure that precede star formation would cause the freeze-out of most carbon- and oxygen containing molecules; with their disappearance, the disruption of N 2 formation pathways in clouds of lesser density would result in a prevalence of gas-phase atomic nitrogen. In turn, this would lead to the efficient production of ammonia and 15 NH 3 / 14 NH 3 ratios higher than the cosmic 15 N/ 14 N ratios (to as much as by 80%).
These predictions are interesting in that they appear to match, albeit in broad terms, the findings in meteorites and the current interpretation of meteoritic amino acid formation. In fact, if the distinctly higher δD values of 2-methyl amino acids seem to point to their syntheses in cold ISM environments and the lower values of 2-H amino acids to suggest that their syntheses took place at a later stage in the presence of liquid water, their δ 15 N opposite trends would also fit with earlier (ISM) and later (prestellar cores) cosmochemical processes, albeit removed from a parent body environment.
Very little is known of the molecular sequence of events that would have taken place in a prestellar core; however, we can expect that several stages of temperature, pressure, and ensuing chemical regimes followed the initial collapse of the presolar portion of the ISM (e.g . , Ceccarelli et al. 2007 ). We could hypothesize, therefore, that some of the warmer stages of star formation might have allowed selected environments, where the desorption, mixing, and reactions of radical, precursor molecules, water, and ammonia led to the syntheses of higher 15 N amino acids and favored shorter molecular species formation. It also appears that such locals and the kinetic processes they allow to envision could, rather than parent body reactions, explain some of the molecular distributions seen in the CR meteorites, such as: the far from unity diastereomer ratios seen for the thermodynamically similar amino acids allo ile and ile ( Chaban and Pizzarello 2007 ), their erratic levels of enantiomeric excesses as well as the preponderance of lower chain length species and the abundance of unreacted carbonyl containing molecules ( Pizzarello and Holmes 2009 ).
Abiotic Pathways to Biomolecules
Meteorites probably present just a minuscule sample of the prebiotic potential of cosmic synthetic processes but, through their studies, we may be able to infer how common or widespread they may be. Transformations of organic compounds, or their synthesis from inorganic compounds, occurs in response to thermodynamic drives, modulated by the kinetic properties of individual reactions. Setting aside the mechanistic details for a moment, it is useful to examine how reactions may or may not be favored by the thermodynamic properties of the system. Reactions involving organic compounds and occurring in aqueous solution may have occurred on meteorite parent bodies, smaller icy aggregates on their way to form asteroids or comets, and in selected prestellar environments; therefore, investigating relative stabilities of aqueous organic compounds may yield clues to these processes. This approach can help to answer specific questions about relative abundances of organic compounds found in carbonaceous meteorites. The following discussion illustrates this approach, with the specific goal of understanding the relative abundances of ammonia, amino acids, and aldehydes.
Stabilities of amino acids relative to other organic compounds during aqueous alteration can be assessed by considering a set of hypothetical overall reactions involving amino acids and other aqueous organic compounds. As an example, the stability of alanine relative to the aldehyde propanal can be assessed by considering a reaction in which carbon is conserved in the two aqueous organic compounds, given by
where the (aq) indicates that the compounds of interest are all dissolved in H 2 O. This reaction is not meant to depict a specific synthetic process, but instead delineates relative stabilities. It is evident from reaction ( 1 ) that there could be abundances of NH 3 (aq) that would favor the stability of alanine relative to propanal. Likewise, at strongly reduced conditions, where there may be considerable H 2 (aq) present, alanine would become unstable relative to propanal and NH 3 (aq). Quantifying the activities (and concentrations) of NH 3 (aq) and H 2 (aq), where such transformations become possible, can be accomplished by considering the equilibrium constant for reaction ( 1 ), and manipulating its law of mass action expression. That expression, in its logarithmic form, is given by
In most dilute aqueous solutions (salinity<seawater, as a rule of thumb), it can be safely assumed that the activity of H 2 O is so close to 1 that setting it equal to 1 introduces only trivial uncertainty. With this assumption, Equation ( 2 ) can be rearranged to give
which represents the equation of a line on a plot of log aNH 3 (aq) vs log aH 2 (aq) with a slope of 2 and an intercept equal to
At constant temperature and pressure, log K is a constant, which means that various lines can be determined based on the activity ratio of alanine to propanal.
Plots of this type are shown in Figure 7 for 0°C and 25°C both at 1 bar, with contours of the activity ratio ranging from 0.001 to 1000. The bold contour labeled 1 in each plot shows the position of equal activities of the two organic solutes at equilibrium. Ranges of relative predominance of propanal and alanine are indicated, with that of alanine in each plot falling at higher activities of NH 3 (aq) and lower activities of H 2 (aq), consistent with Le Chatlier's principle applied to reaction ( 1 ). Also shown in these diagrams are the values of log a H 2 (aq), at which hematite (Fe 2 O 3 ) would be reduced to magnetite (Fe 3 O 4 ), consistent with
and where magnetite would be reduced to the ferrous silicate fayalite (Fe 2 SiO 4 ) in the presence of quartz (SiO 2 ) according to
Magnetite, which is one of the aqueous alteration products identified in CI, CM, CO, CR, CV meteorites and some LL3 chondrites (Zolensky et al. 2008) would be stable between the two vertical dashed lines on each plot.

Equilibrium activity diagrams showing the relative stabilities of aqueous alanine and propanal in terms of the activities of NH 3 (aq) and H 2 (aq) at ( left ) 0°C and 1 bar and ( right ) 25°C and 1 bar. Selected contours of the equilibrium ratio of activities of alanine to propanal from 1000 to 0.001 are indicated. Equilibrium constants for reaction ( 1 ) were calculated with the revised Helgeson-Kirkham-Flowers equation of state ( Shock et al. 1992 ) using data and parameters from Shock et al. (1989) ; Shock and Helgeson (1990) and Shulte and Shock (1993) . Also shown are activities of H 2 (aq) corresponding to equilibrium between hematite and magnetite (HM, reaction 4 ), as well as magnetite, quartz, and fayalite (FMQ, reaction 5 ). Thermodynamic data for minerals come from Helgeson et al. (1978) . All calculations were conducted with the software package SUPCRT92 ( Johnson et al. 1992 ).
The presence of magnetite brackets the equilibrium activities of H 2 (aq) that could have attained during at least a portion of the aqueous alteration processes occurring on the Murchison parent body. If this alteration occurred at 0°C, then the equilibrium activity of H 2 (aq) fell between about 10 −5.2 and 10 −9.1 . If, on the other hand, temperatures were warmer, these activities would change. As an example, at 25°C, the equilibrium activities of H 2 (aq) fall between 10 −4.9 and 10 −8.5 . In dilute solutions, activities of neutral solutes correspond closely to concentrations ( Amend and Shock 2001 ).
These plots reveal the ranges of H 2 (aq) concentrations that are consistent with the occurrence of magnetite, and the NH 3 (aq) concentrations that would provide a thermodynamic drive for the formation of an amino acid rather than an aldehyde, and vice versa . At, for example, log a H 2 (aq)=−7 (equal to about 100 nanomolar dissolved H 2 ), conditions in the middle of the range of magnetite stability, activities of NH 3 (aq)>10 −2 at 0°C, and >10 −3 at 25°C, would favor the formation of alanine at abundances greater than those of propanal. Whether or not equilibrium is actually attained among organic compounds during aqueous alteration events on meteorite parent bodies, the persistent thermodynamic drive to form amino acids or aldehydes depends on the chemical composition of the system. The plots in Figure 7 reveal the quantitative nature of those thermodynamic drives. They also make it possible to begin to understand the amounts of NH 3 (aq) that would be required if amino acid concentrations were similar to aldehyde concentrations or vastly different.
Comparison of the data from the CR2 meteorites and Murchison shown in Figure 4 indicates that the ratio of total amino acids to aldehydes+ketones is on the order of 12 for LAP and about two for GRA and Murchison. It can also be seen that ammonia abundances are greatest in GRA, similarly large in LAP, and very low in Murchison. These data can be combined with the thermodynamic analysis depicted in Figure 7 in an attempt to evaluate what conditions were like during aqueous alteration, if the relative abundances of aldehydes and amino acids were influenced by that stage of meteorite history. It should be kept in mind that the data that exist are for what is present in the meteorites and not what may have been present in aqueous solution during the alteration process. Adsorption equilibria among solutions and various mineral phases may differ for these two classes of organic compounds, and much could have happened to alter ratios inherited from such an early stage in the history of the solar system. Let us assume that the relative abundances of organic compounds in meteorite extracts reflects conditions on the parent bodies at the time the compounds formed and that they were not radically reset by subsequent history.
Starting with LAP, conditions consistent with the overall amino acid to aldehyde ratio would fall just above and to the left of the 10 contour, which is the first above the equal activity (=1) contour in a plot like those shown in Figure 7 for the presently unknown temperature of aqueous alteration. If the amino acid to aldehyde ratio is a result of aqueous alteration, then it provides us with this locus of possibilities in log a NH 3 (aq) versus log a H 2 (aq) space. Likewise, ratios from GRA and Murchison indicate that conditions during aqueous alteration may have generated conditions near or slightly above the equal activity contour. If there were estimates of the activity of either H 2 (aq) or NH 3 (aq) that prevailed during aqueous alteration, then the equilibrium value of the other would be uniquely defined by the ratio of organic compounds.
Assuming that the relative abundances of ammonia in the extracts are analogous to the relative abundances during aqueous alteration leads to the following assessment of relative oxidation-reduction (redox) states during aqueous alteration events. The amino acid to aldehyde ratios in GRA and Murchison are about equal, but the abundance of ammonia that can be extracted from GRA is much greater. Therefore, it seems likely that conditions during aqueous alteration of the Murchison parent body would plot at a lower activity of NH 3 (aq) than those that attained during alteration of the GRA parent body. If so, then the fact that both meteorites fall on about the same contour means that the activity of H 2 (aq) was much greater during alteration of GRA than during alteration of Murchison if alteration processes happened at similar temperatures on both parent bodies. The abundance of ammonia in the LAP extracts is nearly as great as the GRA extracts, but the amino acid to aldehyde ratio is also greater. If the temperature of alteration of LAP was similar to that of GRA, then conditions during the alteration of LAP would fall somewhat lower in log a NH 3 (aq), but also considerably lower in the activity of H 2 (aq) to maintain the higher amino acid to aldehyde ratio. All else being equal, indications are that conditions were most oxidized during alteration of the Murchison parent body, most reduced during alteration of GRA, and intermediate during the alteration of LAP. Corroborating evidence may be found in the relative abundances of carboxylic acids, which are more oxidized than either amino acids or aldehydes. Redox conditions during alteration directly affect the potential for abiotic organic synthesis ( Shock 1990 ; 1992a ; Shock and Schulte 1990 ; 1998 ; Amend and Shock 1998 ; Shock and Canovas 2010 ). If the analysis outlined above survives deeper scrutiny, the overall potential for abiotic organic synthesis from inorganic starting compounds may have been greatest on the GRA parent body, despite its lower abundances of amino acids.
There are several ways that these predictions of relative redox states can be tested. One would be to examine the mineralogy of the alteration products in all three meteorites for evidence of mineral assemblages that could indicate redox conditions that prevailed during alteration. Another would be to seek evidence from mineral assemblages, organic compound associations, and isotopes (oxygen in pairs or suites of minerals that formed together, for example) that could bracket the temperatures of the alteration events on each parent body so that quantitatively appropriate versions of the plots in Figure 7 could be built. Also, experimental studies of the adsorption of ammonia, amino acids, aldehydes, and other organic compounds commonly extracted from meteorites on minerals found in meteorite alteration assemblages would enable estimation of aqueous concentrations or activities from the abundances of these compounds in the meteorites.
Exogenous Delivery and Molecular Evolution
The monomers and their potential.
If we trust the record of impact craters observed in most of solar planets and satellites, meteorites have showered the Earth throughout geological ages and certainly did so soon after its accretion (e.g., Chyba and Sagan 1992 ). Abundant organic materials were just as certainly delivered to the early Earth and, it is reasonable to assume, a good portion of them survived the process. We have learned from the analyses of two largely different types of meteorites that this exogenous input delivered both complex macromolecules of uncertain composition and free soluble compounds. Various molecular species must have interacted in the meteorites already prior to their fall, to a certain degree, because some derivative compounds such as the carboxamides ( Cooper and Cronin 1995 ) are released from their extracts upon hydrolysis; however, peptides have been carefully searched for in the Murchison meteorite and not found, with the exception of diglycine ( Shimoyama and Ogasawara 2002 ). If we are trying to estimate the potential of this delivery for prebiotic evolution and we believe that such evolution had to gain some polymeric complexity for life to ensue, then, we have to conclude that the bulk of meteoritic compounds could have provided, at best, monomeric constituents. In general, however, any evolutionary path has to rely on monomeric material as well and, just comparing with other early planetary processes that could have led to organic compounds such as atmosphere-mediated Miller-Urey-type syntheses or the environment of hydrothermal vents, the molecular species ready-made in meteorites would not appear as too bad of a start. Of these, meteoritic amino acids appear as likely candidates for further molecular evolution, particularly considering their selective and abundant suites found in CR2 chondrites.
Amino acids, the components of extant proteins, are able to polymerize under a variety of laboratory conditions and could have done so in early Earth environments. For example, Oro' and Guidry (1961) first showed that glycine readily polymerizes in the presence of ammonia and little water at temperatures of about 140°C. Also Leman, Orgel, and Ghadiri (2004) showed that the presence of carbonyl sulfide, such as it is found around volcanoes, could lead to easy formation of peptides. When of the type found nonracemic in Murchison, amino acids readily form an activated carboxyl, e.g., as an oxazolone by intramolecular dehydration, and polymerize conforming into helixes at lengths as short as three-amino acid units ( Crisma et al. 2004 ).
These findings suggest that it is plausible that exogenous amino acids acquired at least some polymeric complexity during early terrestrial evolution; it is as likely that their overall molecular properties might have been evolutionary factors as well. For example, all ee found so far for meteoritic amino acids have just one configuration, l -, whereas those obtained for chiral molecules in natural processes, designed experiments, or via theoretical schemes are all subjected to chance outcome in the absence of asymmetric influences. Similarly, several terrestrial crystals such as quartz are chiral but their world-wide production is about equal in d - and l -forms. Also, so far, ee have been found in amino acids that do not racemize 4 , meaning that their ee could have been preserved in prebiotic aqueous environments.
Most importantly, amino acids as well as peptides are molecules with diverse catalytic properties that are readily displayed experimentally and in biochemistry. They can also be asymmetric catalysts, a fact suggesting that the unique molecular asymmetry of meteoritic amino acids might have been a particularly useful evolutionary tool. A set of experiments have been conducted with this theme, to assess the possibility that the nonracemic amino acids of meteorites could have acted as catalysts during early Earth molecular evolution and transferred their asymmetry to other prebiotic building blocks such as sugars. It was found that both amino acids and dipeptides can catalyze the asymmetric aldol condensation of glycolaldehyde, or glycolaldehyde and glyceraldehyde, to produce tetrose ( Pizzarello and Weber 2004 ; Weber and Pizzarello 2006 ) and pentose ( Pizzarello and Weber 2010 ) sugars with significant ee . It is interesting that these syntheses singled out d -erythrose and d -ribose in forming ee with LL dipeptides catalysts, whereas all other sugars acquired either ee of the same configuration as the catalyst or, in some cases, no ee at all. These reactions were conducted in buffered water solution, made use of simple reactant realistically available to the early Earth, and implied likely catalytic pathways under mild conditions. They have, therefore, some prebiotic credibility and support the conclusion that, whereas the extent to which meteoritic catalysts might have been effective in a mixture is entirely unknown, their possible inductive effect toward chiral asymmetry in the monomeric interactions of molecular evolution cannot be disregarded.
Energetic Contingencies
Knowing that life ensued rather quickly in early Earth history, it seems also realistic to assume that the planet environments might have been part of the unknown contingencies that fostered the transition from abiotic chemistry to the molecular evolution that preceded the emergence of life. If so, these environments would have combined available organic compounds with favorable catalysts, which might have been organic as well as inorganic. Just as all known life forms have habitats, the emergence of life may have had a habitat as well ( Shock et al. 1998 , 2000 ).
Because the record of Earth's first geological era was lost to ensuing diagenetic and metamorphic changes, clays represent the first alteration products of basaltic glass under hydrous conditions and would be good candidates for aiding simple abiotic molecules, such as those found in meteorites, in undertaking evolutionary steps of prebiotic significance. As detailed in Deamer and Weber (2010) , these minerals are known to adsorb organic molecules and actively participate as catalysts in their syntheses and reactions (Williams et al. 2005). In particular, the smectite group of expandable clays, such as montmorillonite and saponite, can undergo surface energy changes during diagenesis that will affect their surface H-bonding at key sites and form complex aromatic and polyaromatic hydrocarbons of up to C 20 from methanol (e.g., Williams et al. 2005).
Also, as mentioned above, conditions of very low H 2 O activities or elevated temperatures in aqueous solution can drive polymerization reactions that involve dehydration such as peptide formation. The latter possibility has inspired several experimental investigations of the potential for amino acid polymerization under hydrothermal conditions ( Shock 1992b , 1993 ). Starting with amino acids, it has repeatedly been shown that dipeptides and cyclic dipeptides form rapidly in hydrothermal experiments ( Imai et al. 1999b ; Alargov et al. 2002 ; Li and Brill 2003 ; Lemke et al. 2009 ; Cleaves et al. 2009 ). Occasionally, these experimental studies also obtain small concentrations of tripeptides and longer oligomers ( Imai et al. 1999a ; Tsukahara et al. 2002 ), but the formation of cyclic dipeptides, which is thermodynamically favored ( Shock 1992b ), often dominates. It has also been shown that if experiments are started with somewhat larger oligomers, say three or four amino acids in length, then the peptides can be lengthened by hydrothermal reactions involving the monomers ( Kawamura et al. 2005 ), and that polymers containing up to 20 amino acids can be generated hydrothermally from glutamic acid or aspartic acid, which do not form cyclic dipeptides ( Kawamura and Shimahashi 2008 ). In addition, hydrothermal dehydration reactions involving alkanoic acids and glycerol produce lipid-like molecules capable of self-assembly ( Simoneit et al. 2007 ). Taken together, these recent results show that condensation, polymerization, and peptide bond formation may commonly occur in hydrothermal conditions. If so, planetary processing of materials supplied from meteorites may have been integral to the emergence of living systems.
CHALLENGES AND FUTURE RESEARCH DIRECTIONS
Carbonaceous chondrites are natural samples of limited and unpredicted availability that, once reaching the Earth, are under the constant threat of terrestrial contamination. Their study has obviously met with challenges of material preservation, designing of analytical methodologies, identification of indigenous materials, and more; these will remain, mutatis mutandis , much the same in the future. Nonetheless, these meteorites have been analyzed successfully in great detail and their studies have been invaluable in determining the prebiotic possibilities of cosmochemical environments; however, they have not answered the basic exobiological question of whether extraterrestrial organic compounds contributed to molecular evolution on the early Earth and to the emergence of life.
That answer may never be possible, but, if we believe with Eschenmoser (2008) that life's origin “ … cannot be discovered, as other things in science, it can only be re-invented” , meteorite analyses will offer realistic molecular tools to attempt just that and much still can be done. After forty years of studying Murchison-type meteorites, a new group of Antarctic finds has shown that within the diverse cosmic environments may reside the capabilities of forming organic suites enriched in biomolecule precursors and of high prebiotic appeal. The CR2 organic compounds are still poorly characterized but new studies will define their extent and distribution. Many small molecules that could be useful for initiating molecular evolution could have escaped detection in earlier studies of these pristine meteorites and have not yet been targeted for analyses: glycolaldehyde, glyceraldehydes (detected but not quantified or unpublished), HCN, formamide, urea, and small peptides are all “stuff” required for modeling early evolutionary biology. Hopefully, we shall know soon their distribution in space also.
1 The number represents a classification of petrographic type and estimates asteroidal secondary processes (were 2<1).
2 The acronyms stand for the names of the Antarctica locations where the meteorites were found: Graves mountain and LaPaz ice fields, respectively.
3 The possibility of different stellar nucleosynthetic pathways for the element of nitrogen (e.g., Wannier et al. 1981 ) would also further complicate their interpretation.
4 Racemization, the reversal of configurations in water, involves the loss and reacquisition of hydrogen by the carbon adjacent to the carboxyl group, which is slightly acidic, and is not allowed when the H at C-2 is substituted with a methyl group.
Editors: David Deamer and Jack W. Szostak
Additional Perspectives on The Origins of Life available at www.cshperspectives.org
- Alargov DK, Deguchi S, Tsujii K, Horikoshi K 2002. Reaction behaviors of glycine under super- and subcritical water conditions . Origins Life Evol Biosph 32: 1–12 [ PubMed ] [ Google Scholar ]
- Amend JP, Shock EL 1998. Energetics of amino acid synthesis in hydrothermal ecosystems . Science 281: 1659–1662 [ PubMed ] [ Google Scholar ]
- Amend JP, Shock EL 2001. Energetics of overall metabolic reactions in thermophilic and hyperthermophilic Archaea and Bacteria . FEMS Microbiology Reviews 25: 175–243 [ PubMed ] [ Google Scholar ]
- Arteaga O, Canillas A, Crusats J, El-Achemi Z, Jellison JE, Llorca J, Ribó JM Chiral biases in solids by effect of sheer gradients: A speculation on the deterministic origin of biological homochirality . Orig Life Evol Biosph 40: 27–40 [ PubMed ] [ Google Scholar ]
- Ceccarelli C, Caselli P, Herbst E, Tielens AGGM, Caux E 2007. Extreme deuteration and hot Corinos: The earliest chemical signatures of low-mass star formation . In Protostars and planets (ed. Reipurth VB , Jewitt D, Keil K), pp. 47–62 The University of Arizona Press, Tucson, AZ [ Google Scholar ]
- Chaban G, Pizzarello S 2007. Meteoritic amino acids: The product of still unknown cosmic and solar environments . Abstracts of papers, 39th Lunar and Planetary Conference, Houston, TX Abstract # 1389 [ Google Scholar ]
- Charnley SD, Rodgers SB 2002. The end of interstellar chemistry and the origin of nitrogen in comets and meteorites . A J 569: L133–L137 [ Google Scholar ]
- Chyba CF, Sagan C 1992. Endogenous production, exogenous delivery, and impact-shock synthesis of organic molecules: an inventory for the origins of life . Nature 355: 125–132 [ PubMed ] [ Google Scholar ]
- Cleaves HJ, Aubrey AD, Bada JL 2009. An evaluation of the critical parameters for abiotic peptide synthesis in submarine hydrothermal systems . Origins of Life and the Evolution of the Biosphere 39: 109–126 [ PubMed ] [ Google Scholar ]
- Cooper GW, Cronin JR 1995. Linear and cyclic aliphatic carboxamides of the Murchison meteorite: Hydrolyzable derivatives of amino acids and other carboxylic acids . Geochim Cosmochim Acta 59: 1003–1015 [ PubMed ] [ Google Scholar ]
- Crick FHC, Orgel LE 1973. Directed panspermia . Icarus 19: 341–346 [ Google Scholar ]
- Crisma M, Moretto A, Formaggio F, Kaptein B, Broxterman QB, Toniolo C 2004. Meteoritic C α -methylated α-amino acids an the homochirality of life: Searching for a link . Angew Chem Int Ed 43: 6695–6699 [ PubMed ] [ Google Scholar ]
- Cronin JR, Pizzarello S 1997. Enantiomeric excesses in meteoritic amino acids . Science 275: 951–955 [ PubMed ] [ Google Scholar ]
- Deamer D 1985. Boundary structures are formed by organic components of the Murchison carbonaceous chondrite . Nature 317: 792–794 [ Google Scholar ]
- Deamer D, Weber AL 2010. Bioenergetics and life's origins . Cold Spring Harb Perspect Biol 2: a004929. [ PMC free article ] [ PubMed ] [ Google Scholar ]
- Eschenmoser A 2008. Fundamental questions: An interview with Albert Eschenmoser . In The Scripps Research Institute News and Views : 8 http://www.scripps.edu/newsandviews/e_20080421/ . [ Google Scholar ]
- Helgeson HC, Delany JM, Nesbitt WH, Bird DK 1978. Summary and critique of the thermodynamic properties of rock forming minerals . Am J Sci 278A: 1–229 [ Google Scholar ]
- Imai E-I, Honda H, Hatori K, Brack A, Matsuno K 1999a. Elongation of oligopeptides in a simulated submarine hydrothermal system . Science 283: 831–833 [ PubMed ] [ Google Scholar ]
- Imai E-I, Honda H, Hatori K, Matsuno K 1999b. Autocatalytic synthesis of oligoglycine in a simulated submarine hydrothermal system . Origins of Life and Evolution of the Biosphere 29: 249–259 [ PubMed ] [ Google Scholar ]
- Johnson JW, Oelkers EH, Helgeson HC 1992. SUPCRT92: A software package for calculating the standard molal thermodynamic properties of minerals, gases, aqueous species, and reactions from 1 to 5000 bar and 0 to 1000°C . Computers & Geosciences 18: 932–934 [ Google Scholar ]
- Kawamura K, Shimahashi M 2008. One-step formation of oligopeptide-like molecules from Glu and Asp in hydrothermal environments . Naturwissenschaften 95: 449–454 [ PubMed ] [ Google Scholar ]
- Kawamura K, Nishi T, Sakiyama T 2005. Consecutive elongation of alanine oligopeptides at the second time range under hydrothermal conditions using a microflow reactor system . J Am Chem Soc 127: 522–523 [ PubMed ] [ Google Scholar ]
- Kawasaki T, Hatase K, Fujii Y, Jo K, Soai K, Pizzarello S 2006. The distribution of chiral asymmetry in meteorites: An investigation using asymmetric autocatalytic chiral sensors . Geochim Cosmochim Acta 70: 5395–5402 [ Google Scholar ]
- Lazcano A 2010. Historical development of origins research . Cold Spring Harb Perspect Biol 2: a002089 [ PMC free article ] [ PubMed ] [ Google Scholar ]
- Leman L, Orgel L, Ghadiri MR 2004. Carbonyl sufide-mediated prebiotic formation of peptides . Science 306: 283–286 [ PubMed ] [ Google Scholar ]
- Lemke KH, Rosenbauer RJ, Bird DK 2009. Peptide synthesis in early Earth hydrothermal systems . Astrobiology 9: 141–146 [ PubMed ] [ Google Scholar ]
- Levison HF, Bottke WF, Gounelle M, Morbidelli A, Nesvorn D, Tsiganis K 2009. Contamination of the asteroid belt by primordial trans-Neptunian objects . Nature 460: 364–366 [ PubMed ] [ Google Scholar ]
- Li J, Brill TB 2003. Spectroscopy of hydrothermal reactions. 27. Simultaneous determination of hydrolysis rate constants of glycylglycine to glycine and glycylglycine-diketopiperazine equilibrium constants at 310-330°C and 275 bar . J Phys Chem A 107: 8575–8577 [ Google Scholar ]
- Loinard S, Castets A, Ceccarelli C, Tielens AGGM, Faure A, Caux E, Duvert G 2000. Double deuterated molecular species in protostellar environments . Astron Astrophys 359: 1169–1174 [ Google Scholar ]
- Luisi PL 2007. Presented at: International School of Complexity-4th course: Basic questions on the Origin of Life ; Ettore Majorana Foundation and Centre for Scientific Culture, Erice, Italy, 1–6 October 2006 [ Google Scholar ]
- Mautner MN, Leonard R, Deamer DW 1995. Meteorite organics in planetary environments: Hydrothermal release, surface activity and microbial utilization . Planet Space Sci 43: 139–147 [ PubMed ] [ Google Scholar ]
- Miller SL, Urey HC, Oró J 1976. Origin of organic compounds on the primitive earth in meteorites . J Mol Evol 9: 59–72 [ PubMed ] [ Google Scholar ]
- Oro' J, Guidry CL 1961. Direct synthesis of polypeptide I. Polycondensation of glycine in aqueous ammonia . Arch Biochem Biophys 93: 166–171 [ PubMed ] [ Google Scholar ]
- Pearson VK, Kearsley AT, Sephton MA, Gilmour I 2007. The labelling of meteoritic organic material using osmium tetroxide vapour impregnation . Planet Space Sci 55: 1310–1318 [ Google Scholar ]
- Peltzer ET, Bada JL 1978. α-Hydroxycarboxylic acids in the Murchison meteorite . Nature 272: 443–444 [ Google Scholar ]
- Pizzarello S. The chemistry that preceded life's origin: When is an evolutionary story an emergent story? Orig Life Evol Biosph. 2009 (in press) [ Google Scholar ]
- Pizzarello S, Cooper GW 2001. Molecular and chiral analyses of some protein amino acid derivatives in the Murchison and Murray meteorites . Meteorit Planet Sci 36: 897–909 [ Google Scholar ]
- Pizzarello S, Holmes W 2009. Nitrogen-containing compounds in two CR2 meteorites: 15 N composition, molecular distribution and precursor molecules . Geochim Cosmochim Acta 73: 2150–2162 [ Google Scholar ]
- Pizzarello S, Huang Y 2005. The deuterium enrichment of individual amino acids in carbonaceous meteorites: A case for the presolar distribution of biomolecules precursors . Geochim Cosmochim Acta 69: 599–605 [ Google Scholar ]
- Pizzarello S, Weber AL 2004. Prebiotic amino acids as asymmetric catalysts . Science 303: 1151. [ PubMed ] [ Google Scholar ]
- Pizzarello S, Weber AL 2010. Stereoselective syntheses of pentose sugars under realistic prebiotic conditions . Orig Life Evol Biosph 40: 3–10 [ PubMed ] [ Google Scholar ]
- Pizzarello S, Zolensky M, Turk KA 2003. Non racemic isovaline in the Murchison meteorite: chiral distribution and mineral association . Geochim Cosmochim Acta 67: 1589–1595 [ Google Scholar ]
- Pizzarello S, Huang Y, Alexandre MR 2008. Molecular asymmetry in extraterrestrial chemistry: Insights from a pristine meteorite . Proc Natl Acad Sci 105: 3700–3704 [ PMC free article ] [ PubMed ] [ Google Scholar ]
- Rodgers SB, Charnley SB 2004. Interstellar diazenylium recombination and nitrogen isotopic fractionation . Mont Not R Astron Soc 352: 600–604 [ Google Scholar ]
- Rodgers SB, Charnley SB 2008. Nitrogen superfractionation in dense cloud cores . Mont Not R Astron Soc 569: L48–L52 [ Google Scholar ]
- Roueff E, Gerin M 2003. Deuterium in molecules of the interstellar medium . Space Scie Rev 106: 61–72 [ Google Scholar ]
- Shimoyama A, Ogasawara R 2002. Dipeptides and diketopiperazines in the Yamato-791198 and Murchison carbonaceous chondrites . Orig Life Evol Biosph 32: 165–179 [ PubMed ] [ Google Scholar ]
- Shock EL 1990. Geochemical constraints on the origin of organic compounds in hydrothermal systems . Origins Life Evol Biosph 20: 331–367 [ PubMed ] [ Google Scholar ]
- Shock EL 1992a. Chemical environments in submarine hydrothermal systems . In: Marine hydrothermal systems and the origin of life , (ed. Holm N) a special issue of Origins Life Evol Biosph 22: 67–107 [ PubMed ] [ Google Scholar ]
- Shock EL 1992b. Stability of peptides in high temperature aqueous solutions . Geochim Cosmochim Acta 56: 3481–3491 [ Google Scholar ]
- Shock EL 1993. Hydrothermal dehydration of aqueous organic compounds . Geochim Cosmochim Acta 57: 3341–3349 [ Google Scholar ]
- Shock EL, Canovas P 2010. The potential for abiotic organic synthesis and biosynthesis at seafloor hydrothermal systems . Geofluids (in press) [ Google Scholar ]
- Shock EL, Helgeson HC 1990. Calculation of the thermodynamic and transport properties of aqueous species at high pressures and temperatures: Standard partial molal properties of organic species . Geochim Cosmochim Acta 54: 915–945 [ Google Scholar ]
- Shock EL, Schulte MD 1990. Amino acid synthesis in carbonaceous meteorites by aqueous alteration of polycyclic aromatic hydrocarbons . Nature 343: 728–731 [ PubMed ] [ Google Scholar ]
- Shock EL, Schulte MD 1998. Organic synthesis during fluid mixing in hydrothermal systems . J Geophys Res 103: 28513–28527 [ Google Scholar ]
- Shock EL, Amend JP, Zolotov MYu 2000. The early Earth vs. the origin of life . In The origin of the Earth and Moon (ed. Canup R, Righter K), pp. 527–543 University of Arizona Press [ Google Scholar ]
- Shock EL, Helgeson HC, Sverjensky DA 1989 Calculation of the thermodynamic and transport properties of aqueous species at high pressures and temperatures: Standard partial molal properties of inorganic neutral species . Geochim Cosmochim Acta 53: 2157–2183 [ Google Scholar ]
- Shock EL, McCollom T, Schulte MD 1998 The emergence of metabolism from within hydrothermal systems . In Thermophiles: The keys to molecular evolution and the origin of life? (ed. Adams Wiegel), pp. 59–76 Taylor & Francis, London, UK [ Google Scholar ]
- Shock EL, Oelkers EH, Johnson JW, Sverjensky DA, Helgeson HC 1992. Calculation of the thermodynamic properties of aqueous species at high pressures and temperatures: Effective electrostatic radii, dissociation constants, and standard partial molal properties to 1000°C and 5 kb . J Chem Soc, Faraday Trans 88: 803–826 [ Google Scholar ]
- Shulte MD, Shock EL 1993. Aldehydes in hydrothermal solutions: Standard partial molal thermodynamic properties and relative stabiirties at high-temperatures and pressures . Geochim Cosmochim Acta 57: 3835–3846 [ PubMed ] [ Google Scholar ]
- Simoneit BRT, Rushdi AI, Deamer DW 2007. Abiotic formation of acylglycerols under simulated hydrothermal conditions and self-assembly properties of such lipid products . Adv Space Res 40: 1649–1656 [ Google Scholar ]
- Tsukahara K, Imai E-I, Honda H, Hatori K, Matsuno K 2002. Prebiotic oligomerization on or inside lipid vesicles in hydrothermal environments . Origins Life Evol Biosph 32: 13–21 [ PubMed ] [ Google Scholar ]
- Wannier PG, Linke RA, Penzias AA 1981. Observations of N-14N-15 in the galactic disk . Ap J 247: 522–529 [ Google Scholar ]
- Weber AL, Pizzarello S 2006. The peptide catalyzed stereospecific synthesis of tetroses: A possible model for prebiotic molecular evolution . Proc Natl Acad Sci 103: 12713–12717 [ PMC free article ] [ PubMed ] [ Google Scholar ]
- Yabuta H, Wiliams LB, Cody GD, Alexander CMO'D, Pizzarello S 2007. The insoluble carbonaceous material of CM chondrites: A possible source of discrete organic compounds under hydrothermal conditions . Meteor Planet Sci 42: 37–48 [ Google Scholar ]
If you're seeing this message, it means we're having trouble loading external resources on our website.
If you're behind a web filter, please make sure that the domains *.kastatic.org and *.kasandbox.org are unblocked.
To log in and use all the features of Khan Academy, please enable JavaScript in your browser.
AP®︎/College Biology
Course: ap®︎/college biology > unit 7.
- Earth formation
- Beginnings of life
- Origins of life
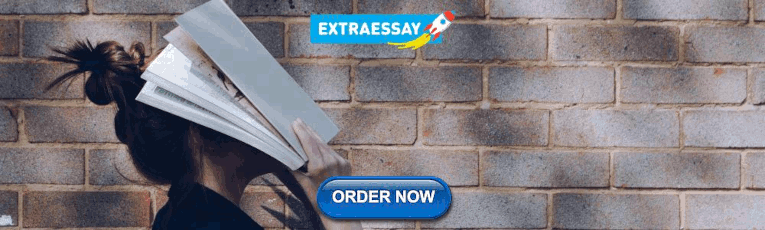
Hypotheses about the origins of life
- The RNA origin of life
- Origins of life on Earth

Key points:
- The Earth formed roughly 4.5 billion years ago, and life probably began between 3.5 and 3.9 billion years ago.
- The Oparin-Haldane hypothesis suggests that life arose gradually from inorganic molecules, with “building blocks” like amino acids forming first and then combining to make complex polymers.
- The Miller-Urey experiment provided the first evidence that organic molecules needed for life could be formed from inorganic components.
- Some scientists support the RNA world hypothesis , which suggests that the first life was self-replicating RNA. Others favor the metabolism-first hypothesis , placing metabolic networks before DNA or RNA.
- Simple organic compounds might have come to early Earth on meteorites.
Introduction
When did life appear on earth, the earliest fossil evidence of life, how might life have arisen.
- Simple inorganic molecules could have reacted (with energy from lightning or the sun) to form building blocks like amino acids and nucleotides, which could have accumulated in the oceans, making a "primordial soup." 3
- The building blocks could have combined in further reactions, forming larger, more complex molecules (polymers) like proteins and nucleic acids, perhaps in pools at the water's edge.
- The polymers could have assembled into units or structures that were capable of sustaining and replicating themselves. Oparin thought these might have been “colonies” of proteins clustered together to carry out metabolism, while Haldane suggested that macromolecules became enclosed in membranes to make cell-like structures 4 , 5 .
From inorganic compounds to building blocks
Were miller and urey's results meaningful, from building blocks to polymers, what was the nature of the earliest life, the "genes-first" hypothesis, the "metabolism-first" hypothesis, what might early cells have looked like, another possibility: organic molecules from outer space.
- Miller, Urey, and others showed that simple inorganic molecules could combine to form the organic building blocks required for life as we know it.
- Once formed, these building blocks could have come together to form polymers such as proteins or RNA.
- Many scientists favor the RNA world hypothesis, in which RNA, not DNA, was the first genetic molecule of life on Earth. Other ideas include the pre-RNA world hypothesis and the metabolism-first hypothesis.
- Organic compounds could have been delivered to early Earth by meteorites and other celestial objects.
Works cited:
- Harwood, R. (2012). Patterns in palaeontology: The first 3 billion years of evolution. Palaeontology , 2(11), 1-22. Retrieved from http://www.palaeontologyonline.com/articles/2012/patterns-in-palaeontology-the-first-3-billion-years-of-evolution/ .
- Wacey, D., Kilburn, M. R., Saunders, M., Cliff, J., and Brasier, M. D. (2011). Microfossils of sulphur-metabolizing cells in 3.4-billion-year-old rocks of Western Australia. Nature Geoscience , 4 , 698-702. http://dx.doi.org/10.1038/ngeo1238 .
- Primordial soup. (2016, January 20). Retrieved May 22, 2016 from Wikipedia: https://en.wikipedia.org/wiki/Primordial_soup .
- Gordon-Smith, C. (2003). The Oparin-Haldane hypothesis. In Origin of life: Twentieth century landmarks . Retrieved from http://www.simsoup.info/Origin_Landmarks_Oparin_Haldane.html .
- The Oparin-Haldane hypothesis. (2015, June 14). In Structural biochemistry . Retrieved May 22, 2016 from Wikibooks: https://en.wikibooks.org/wiki/Structural_Biochemistry/The_Oparin-Haldane_Hypothesis .
- Kimball, J. W. (2015, May 17). Miller's experiment. In Kimball's biology pages . Retrieved from http://www.biology-pages.info/A/AbioticSynthesis.html#Miller's_Experiment .
- Earth’s early atmosphere. (Dec 2, 2011). In Astrobiology Magazine . Retrieved from http://www.astrobio.net/topic/solar-system/earth/geology/earths-early-atmosphere/ .
- McCollom, T. M. (2013). Miller-Urey and beyond: What have learned about prebiotic organic synthesis reactions in the past 60 years? Annual Review of Earth and Planetary Sciences , 41_, 207-229. http://dx.doi.org/10.1146/annurev-earth-040610-133457 .
- Powner, M. W., Gerland, B., and Sutherland, J. D. (2009). Synthesis of activated pyrimidine ribonucleotides in prebiotically plausible conditions. Nature , 459 , 239-242. http://dx.doi.org/10.1038/nature08013 .
- Lurquin, P. F. (June 5, 2003). Proteins and metabolism first: The iron-sulfur world. In The origins of life and the universe (pp. 110-111). New York, NY: Columbia University Press.
- Ferris, J. P. (2006). Montmorillonite-catalysed formation of RNA oligomers: The possible role of catalysis in the origins of life. Philos. Trans. R. Soc. Lond. B. Bio.l Sci ., 361 (1474), 1777–1786. http://dx.doi.org/10.1098/rstb.2006.1903 .
- Kimball, J. W. (2015, May 17). Assembling polymers. In Kimball's biology pages . Retrieved from
- Montmorillonite. (2016, 28 March). Retrieved May 22, 2016 from Wikipedia: https://en.wikipedia.org/wiki/Montmorillonite .
- Wilkin, D. and Akre, B. (2016, March 23). First organic molecules - Advanced. In CK-12 biology advanced concepts . Retrieved from http://www.ck12.org/book/CK-12-Biology-Advanced-Concepts/section/10.8/ .
- Hollenstein, M. (2015). DNA catalysis: The chemical repertoire of DNAzymes. Molecules , 20 (11), 20777–20804. http://dx.doi.org/10.3390/molecules201119730 .
- Breaker, R. R. and Joyce, G. F. (2014). The expanding view of RNA and DNA function. Chemistry & biology , 21 (9), 1059–1065. http://dx.doi.org/10.1016/j.chembiol.2014.07.008 .
- Alberts, B., Johnson, A., Lewis, J., Raff, M., Roberts, K., and Walter, P. (2002). A pre-RNA world probably predates the RNA world. In Molecular biology of the cell (4th ed.). New York, NY: Garland Science. Retrieved from http://www.ncbi.nlm.nih.gov/books/NBK26876/#_A1124_ .
- Moran, L. A. (2009, May 15). Metabolism first and the origin of life. In Sandwalk: Strolling with a skeptical biochemist . Retrieved from http://www.simsoup.info/Origin_Landmarks_Oparin_Haldane.html .
- Kimball, J. W. (2015, May 17). The first cell? In Kimball's biology pages . Retrieved from http://www.biology-pages.info/A/AbioticSynthesis.html#TheFirstCell?
- Kimball, J. W. (2015, May 17). Molecules from outer space? In Kimball's biology pages . Retrieved from http://www.biology-pages.info/A/AbioticSynthesis.html#Molecules_from_outer_space? .
- Jeffs, W. (2006, November 30). NASA scientists find primordial organic matter in meteorite. In NASA news . Retrieved from http://www.nasa.gov/centers/johnson/news/releases/2006/J06-103.html .
Additional references:
Want to join the conversation.
- Upvote Button navigates to signup page
- Downvote Button navigates to signup page
- Flag Button navigates to signup page

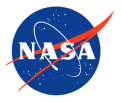
Suggested Searches
- Climate Change
- Expedition 64
- Mars perseverance
- SpaceX Crew-2
- International Space Station
- View All Topics A-Z
Humans in Space
Earth & climate, the solar system, the universe, aeronautics, learning resources, news & events.
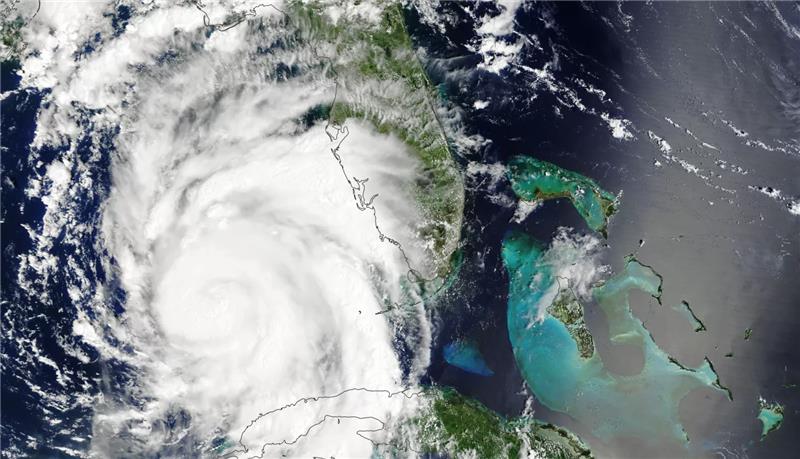
NASA, IBM Research to Release New AI Model for Weather, Climate
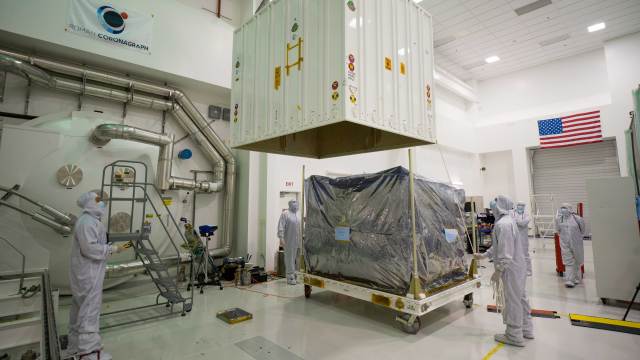
NASA Tool Gets Ready to Image Faraway Planets
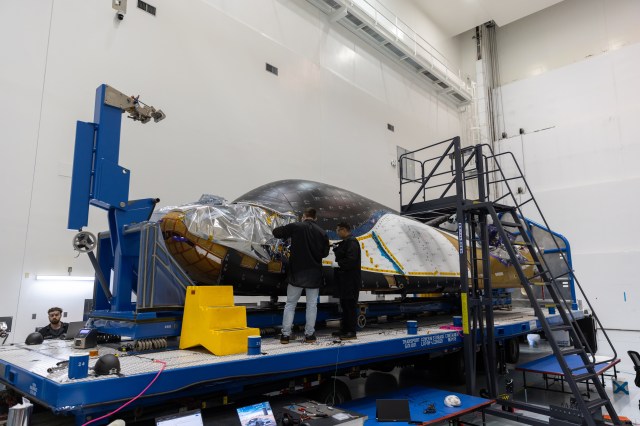
NASA, Sierra Space Deliver Dream Chaser to Florida for Launch Preparation
- Search All NASA Missions
- A to Z List of Missions
- Upcoming Launches and Landings
- Spaceships and Rockets
- Communicating with Missions
- James Webb Space Telescope
- Hubble Space Telescope
- Why Go to Space
- Astronauts Home
- Commercial Space
- Destinations
- Living in Space
- Explore Earth Science
- Earth, Our Planet
- Earth Science in Action
- Earth Multimedia
- Earth Science Researchers
- Pluto & Dwarf Planets
- Asteroids, Comets & Meteors
- The Kuiper Belt
- The Oort Cloud
- Skywatching
- The Search for Life in the Universe
- Black Holes
- The Big Bang
- Dark Energy & Dark Matter
- Earth Science
- Planetary Science
- Astrophysics & Space Science
- The Sun & Heliophysics
- Biological & Physical Sciences
- Lunar Science
- Citizen Science
- Astromaterials
- Aeronautics Research
- Human Space Travel Research
- Science in the Air
- NASA Aircraft
- Flight Innovation
- Supersonic Flight
- Air Traffic Solutions
- Green Aviation Tech
- Drones & You
- Technology Transfer & Spinoffs
- Space Travel Technology
- Technology Living in Space
- Manufacturing and Materials
- Science Instruments
- For Kids and Students
- For Educators
- For Colleges and Universities
- For Professionals
- Science for Everyone
- Requests for Exhibits, Artifacts, or Speakers
- STEM Engagement at NASA
- NASA's Impacts
- Centers and Facilities
- Directorates
- Organizations
- People of NASA
- Internships
- Our History
- Doing Business with NASA
- Get Involved
- Aeronáutica
- Ciencias Terrestres
- Sistema Solar
- All NASA News
- Video Series on NASA+
- Newsletters
- Social Media
- Media Resources
- Upcoming Launches & Landings
- Virtual Events
- Sounds and Ringtones
- Interactives
- STEM Multimedia
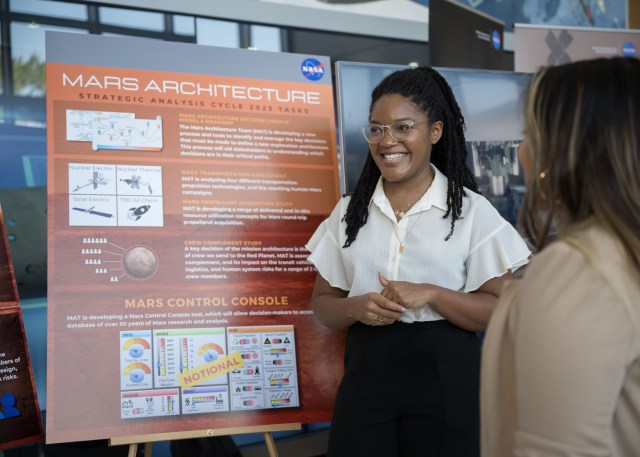
Clare Luckey: Shaping the Future of Mars Missions and Inspiring the Artemis Generation
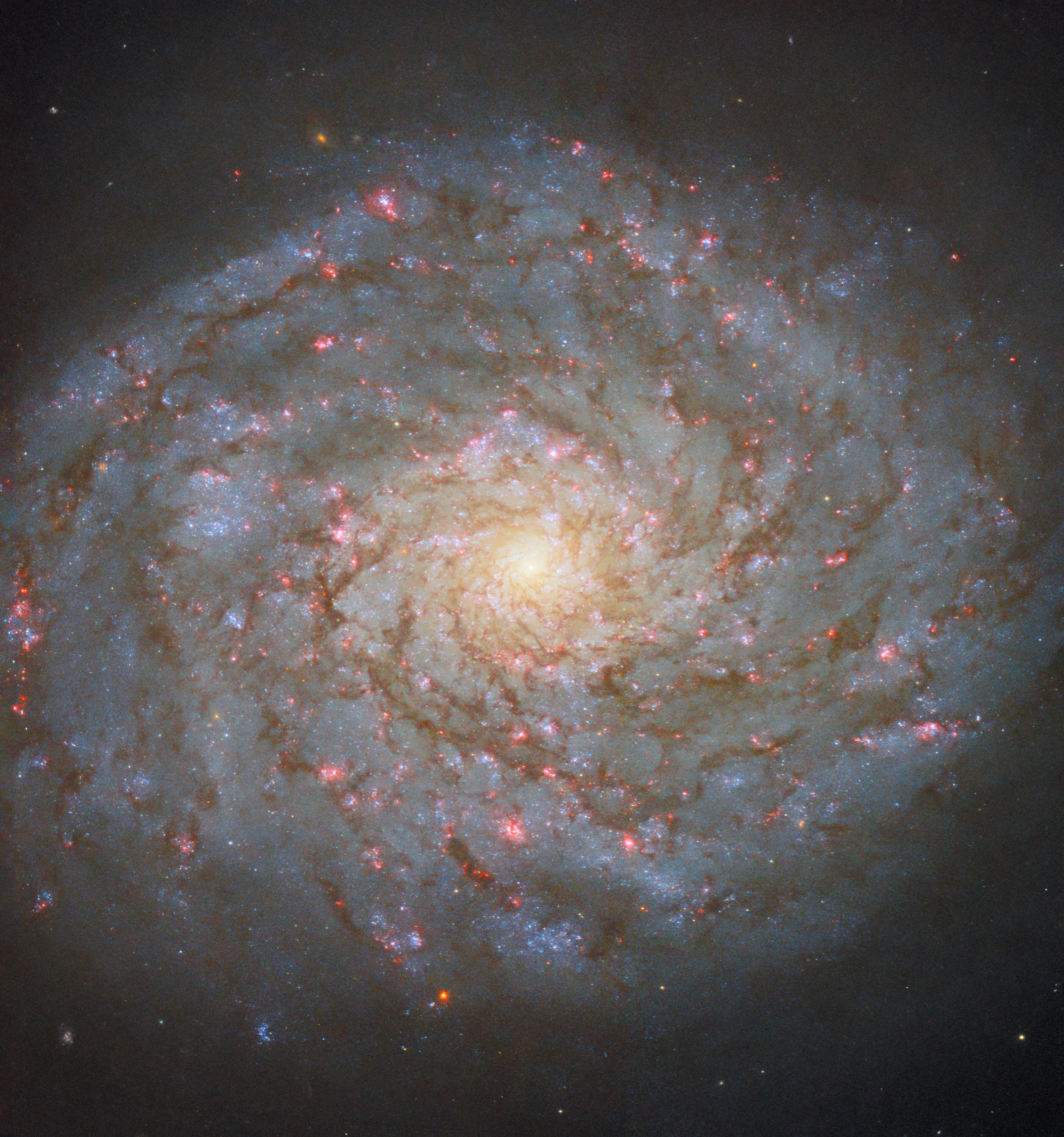
Hubble Captures a Bright Spiral in the Queen’s Hair
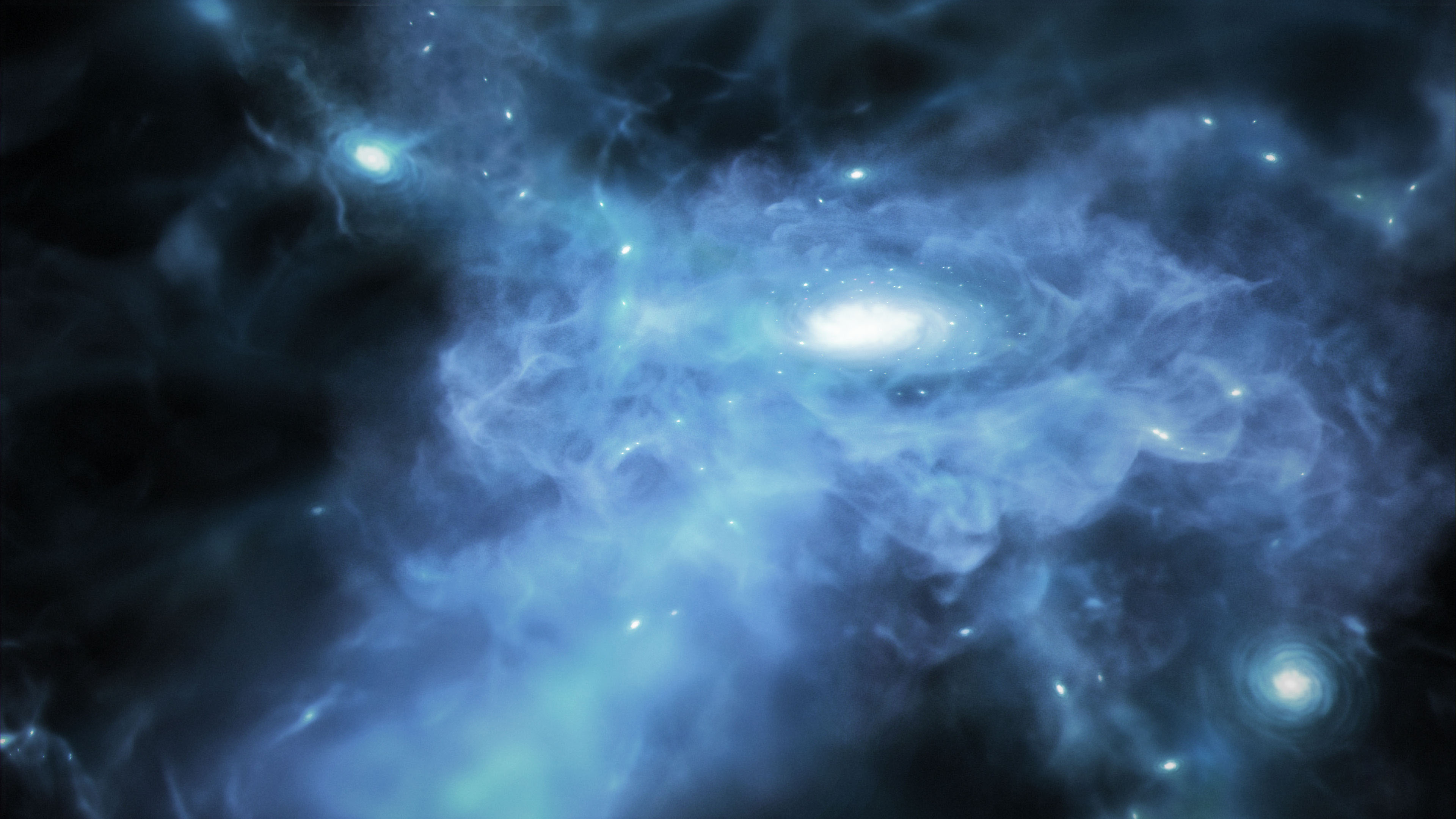
Galaxies Actively Forming in Early Universe Caught Feeding on Cold Gas
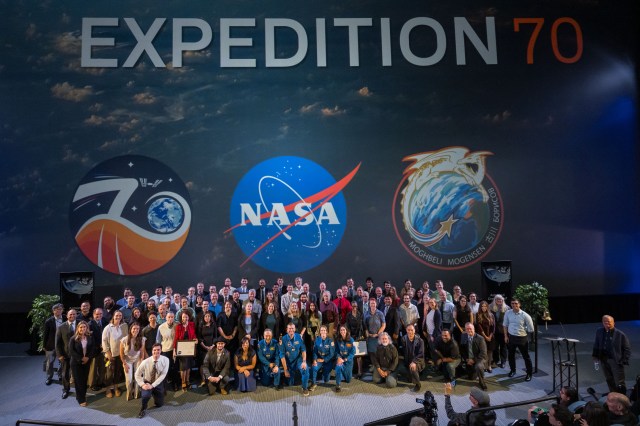
Welcome Back to Planet Earth, Expedition 70 Crew!
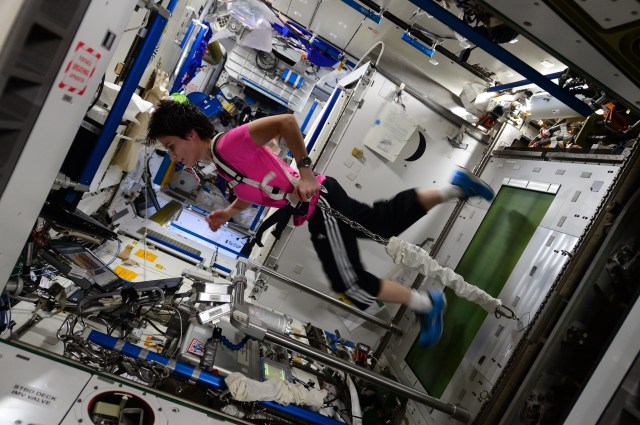
Astronaut Exercise
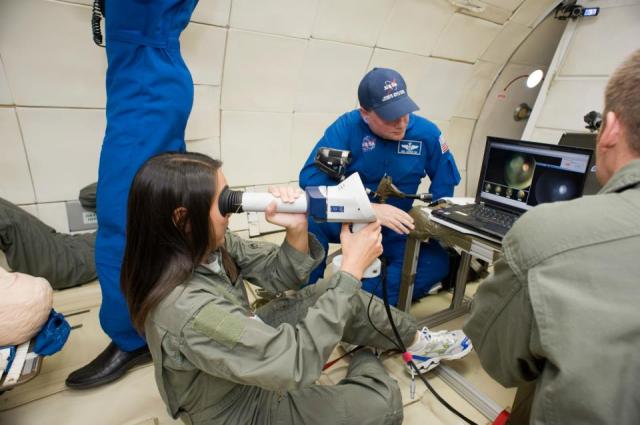
Eleasa Kim: Pioneering CLDP Payload Operations and Cultural Integration
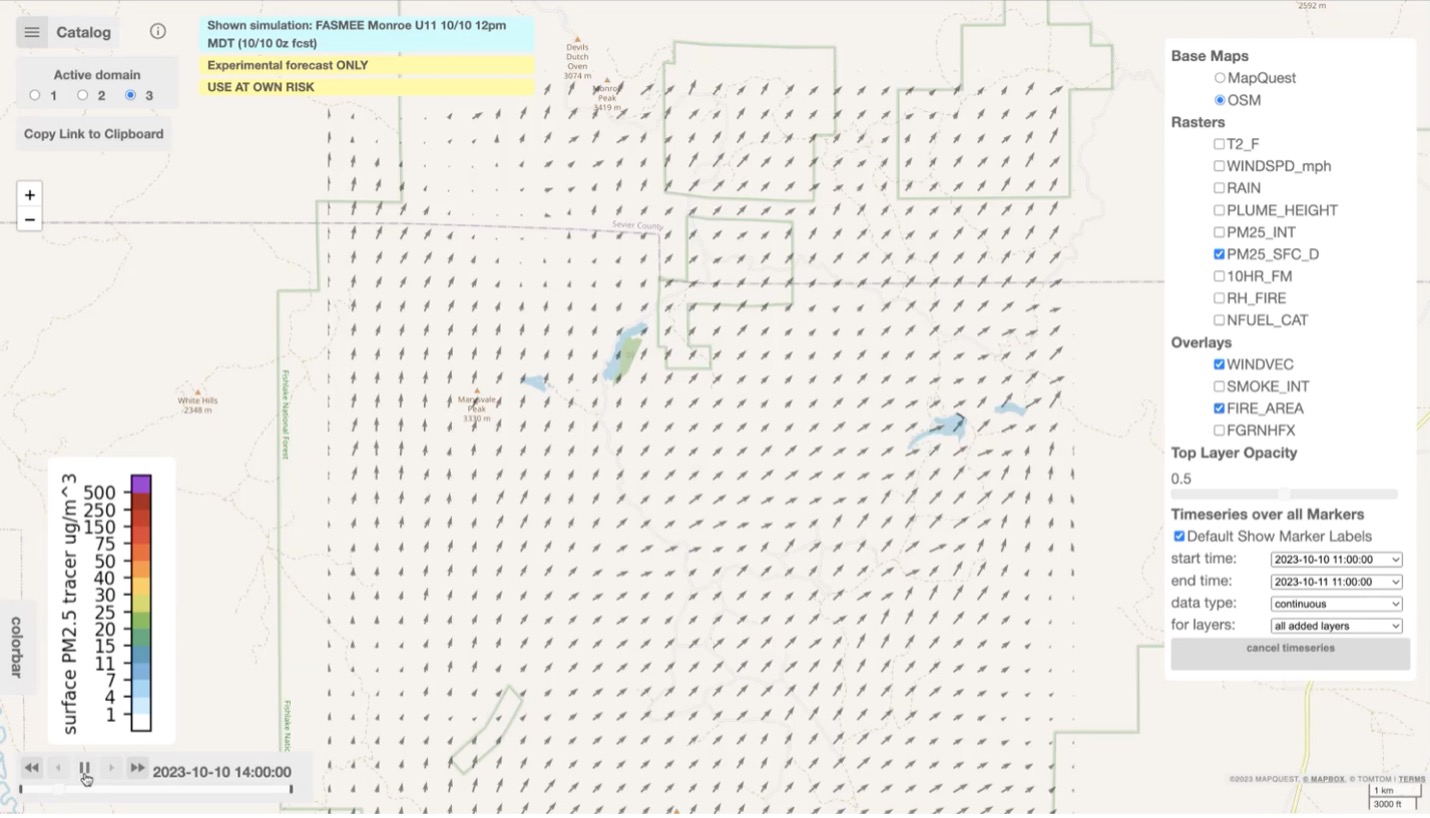
NASA “Wildfire Digital Twin” Pioneers New AI Models and Streaming Data Techniques for Forecasting Fire and Smoke
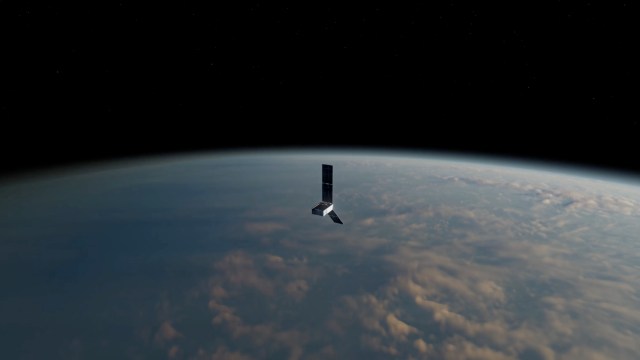
5 Things to Know About NASA’s Tiny Twin Polar Satellites
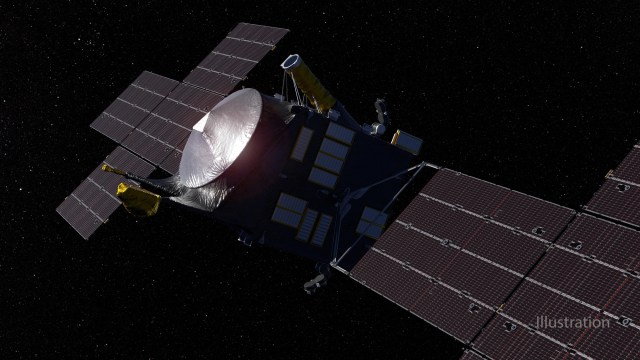
NASA’s Psyche Fires Up Its Sci-Fi-Worthy Thrusters
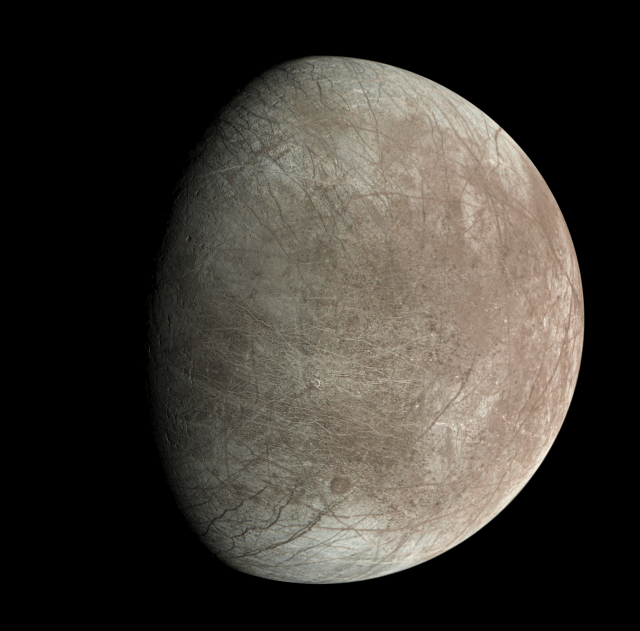
NASA’s Juno Provides High-Definition Views of Europa’s Icy Shell
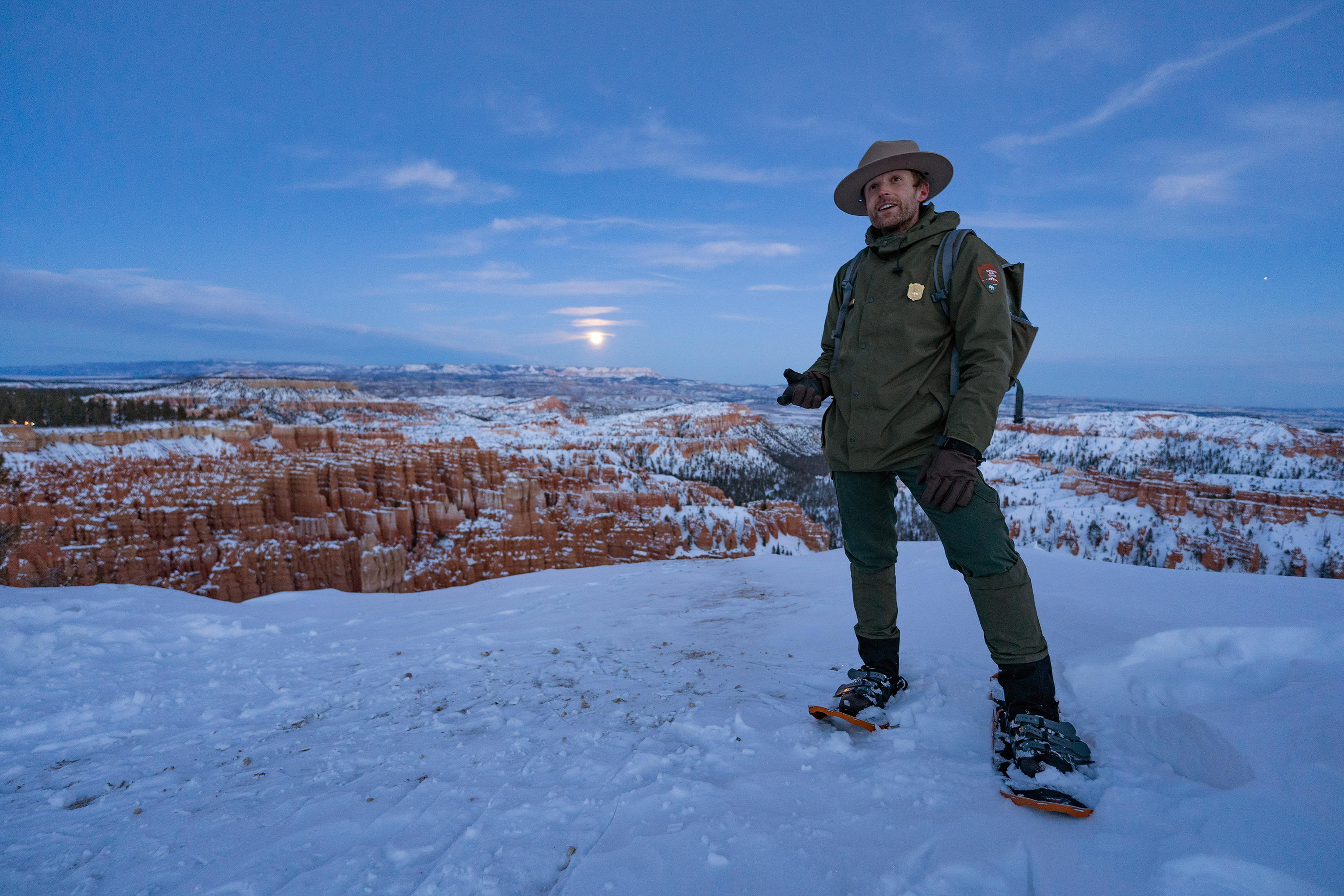
The Next Full Moon is the Flower, Corn, or Corn Planting Moon
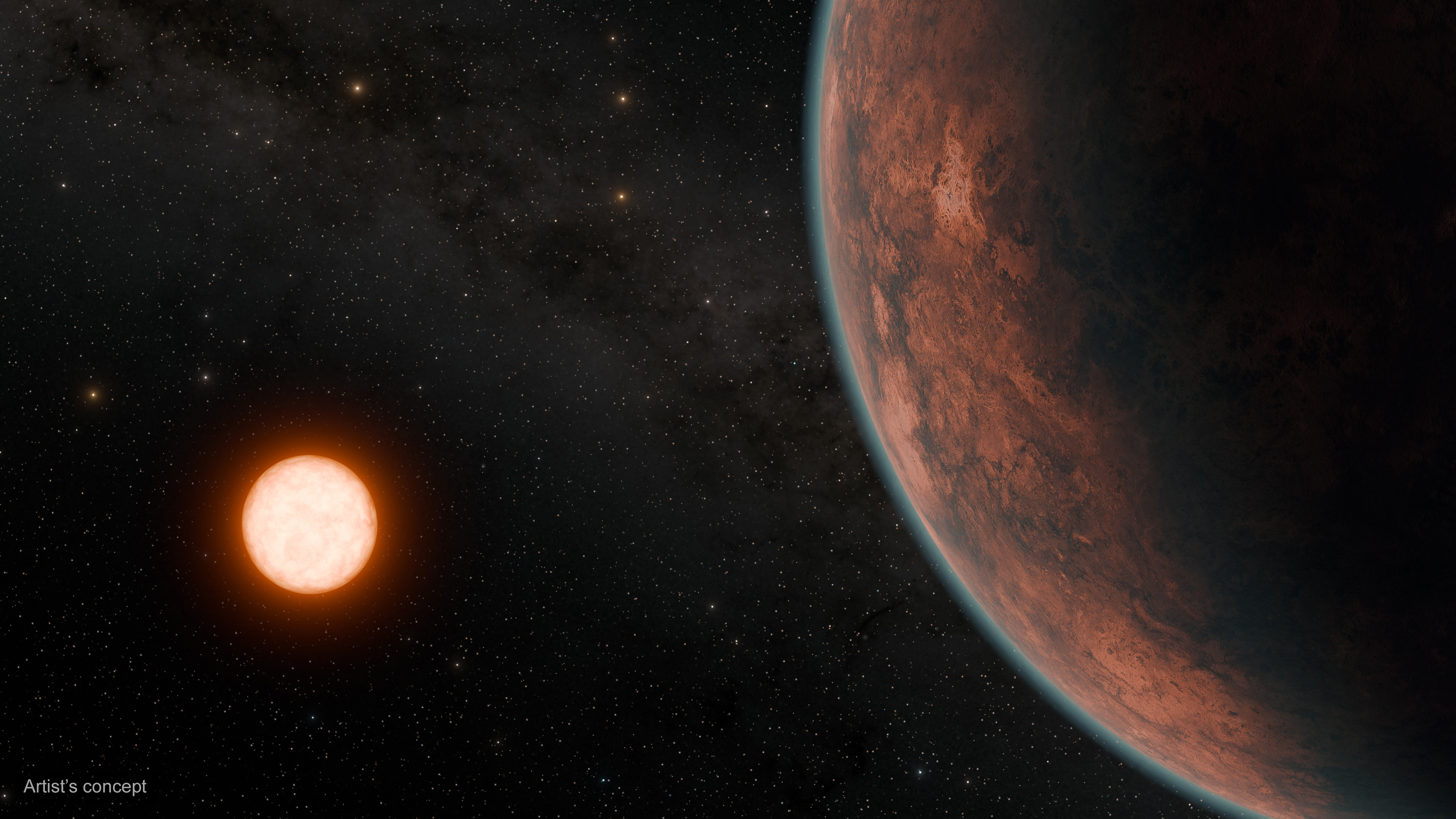
NASA’s TESS Finds Intriguing World Sized Between Earth, Venus
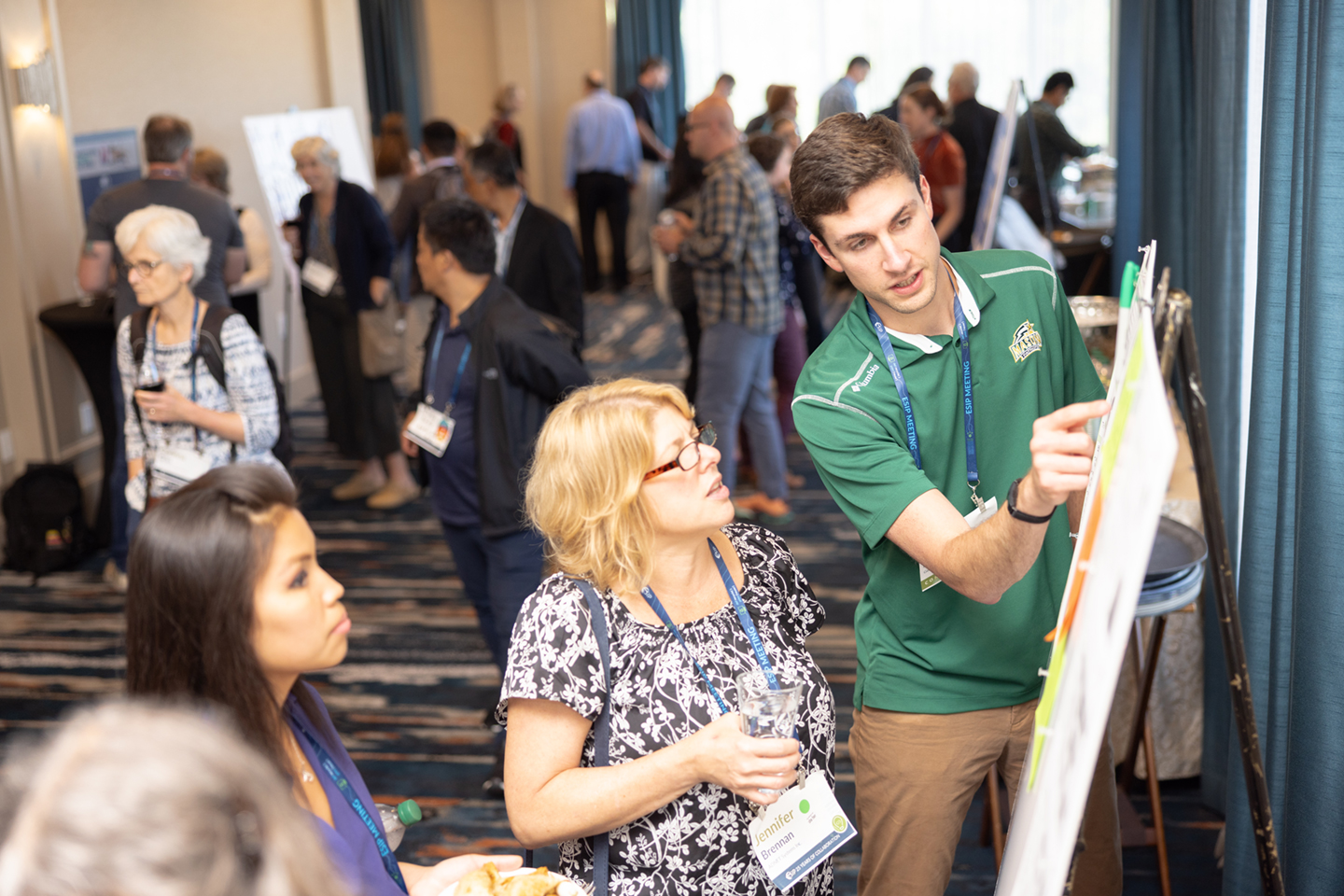
Open Science News
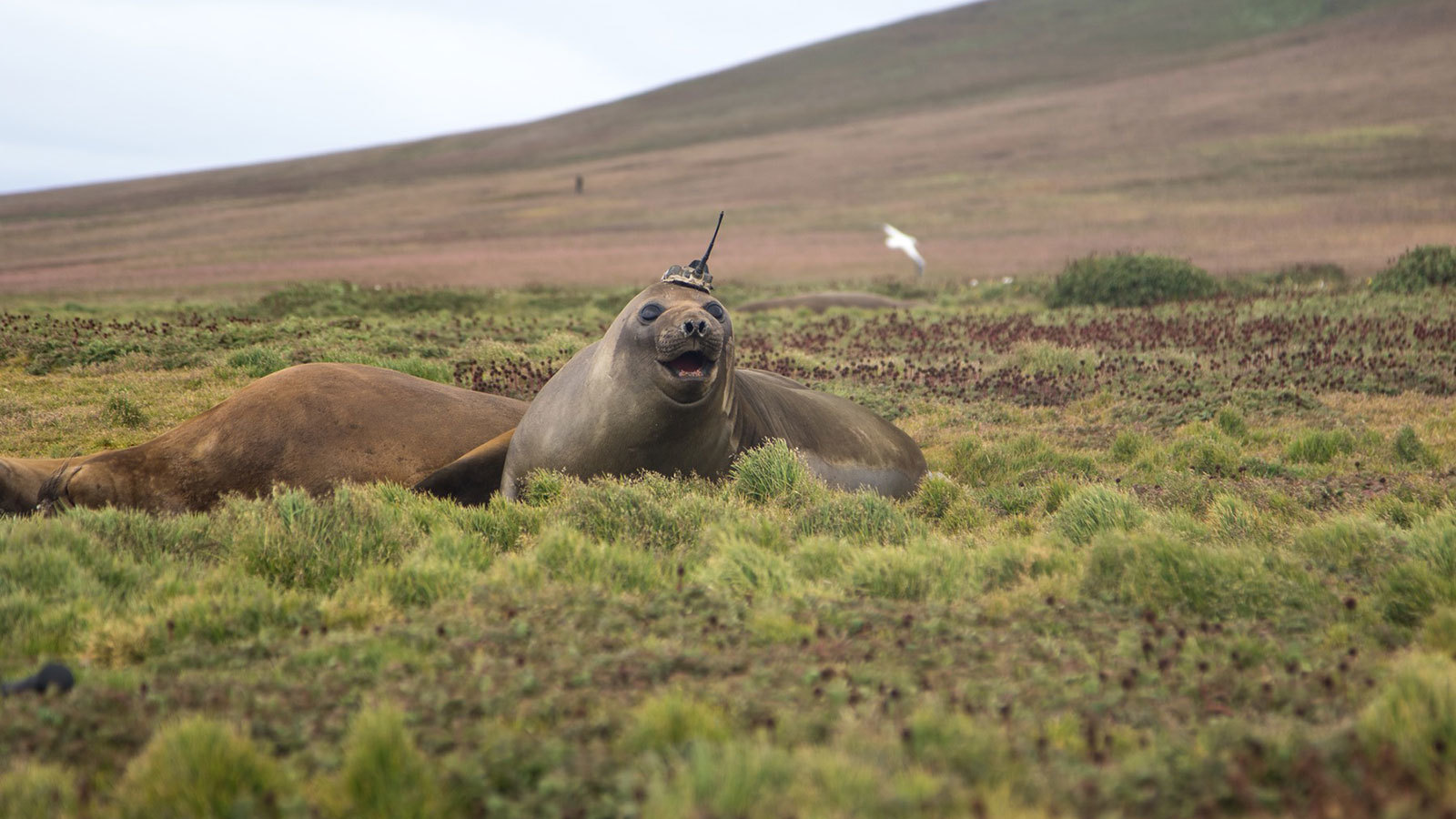
E.11 Consortium in Biological Sciences Clarification on how to Address Eligibility
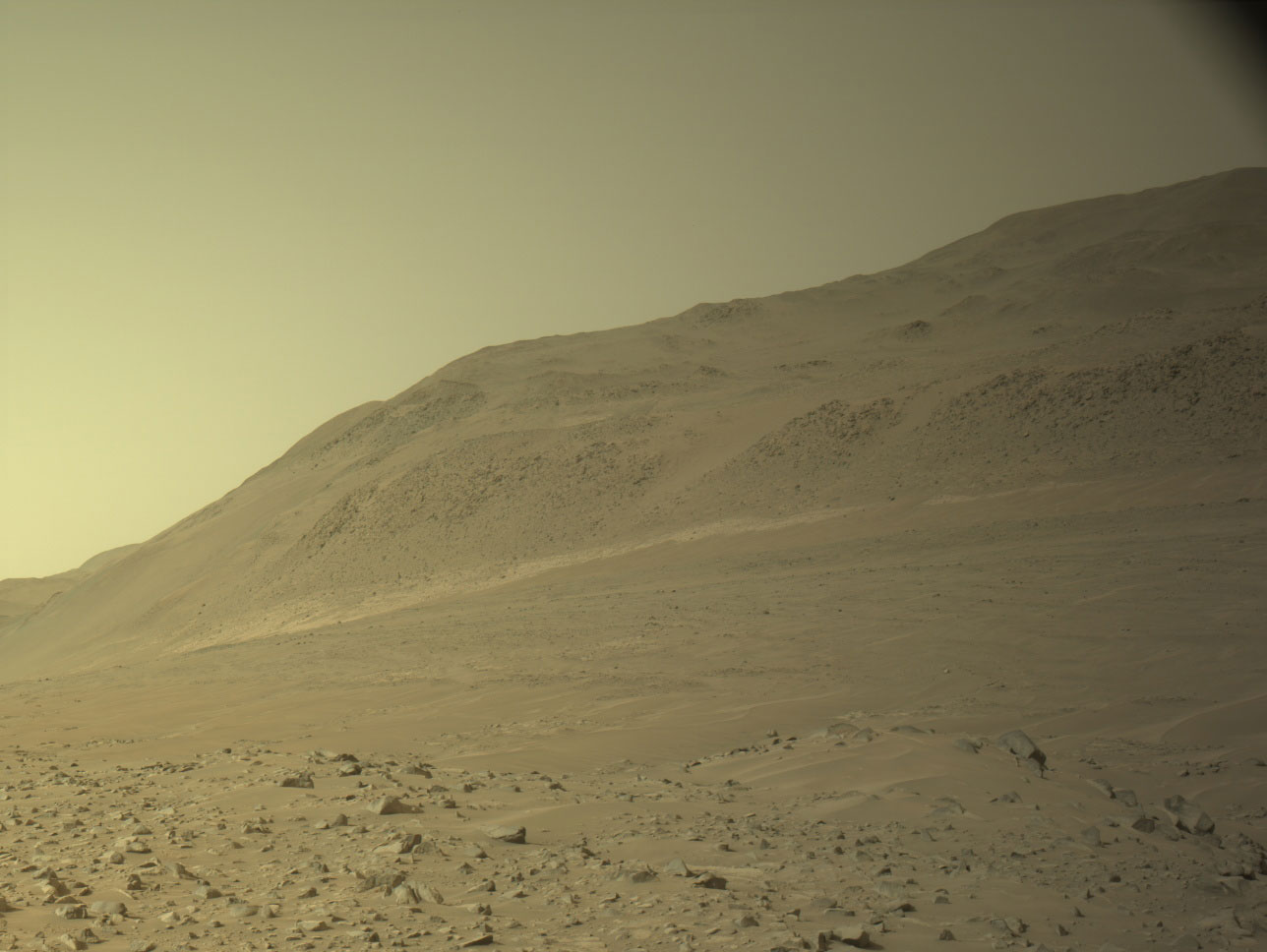
Updated Points of Contact for LDAP, MDAP, and DDAP
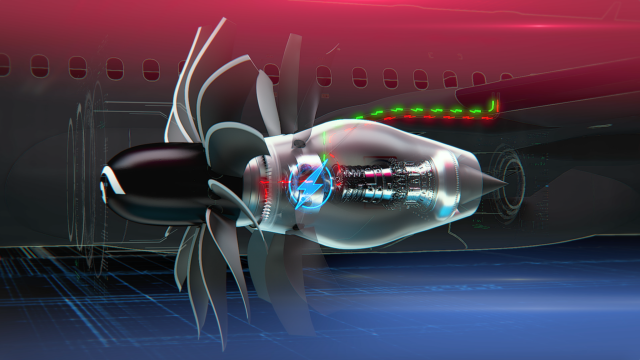
NASA, Industry to Start Designing More Sustainable Jet Engine Core
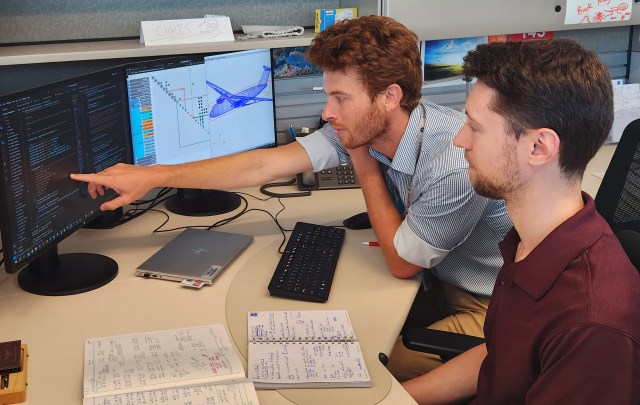
Aviary: A New NASA Software Platform for Aircraft Modelling

NASA’s X-59 Passes Milestone Toward Safe First Flight
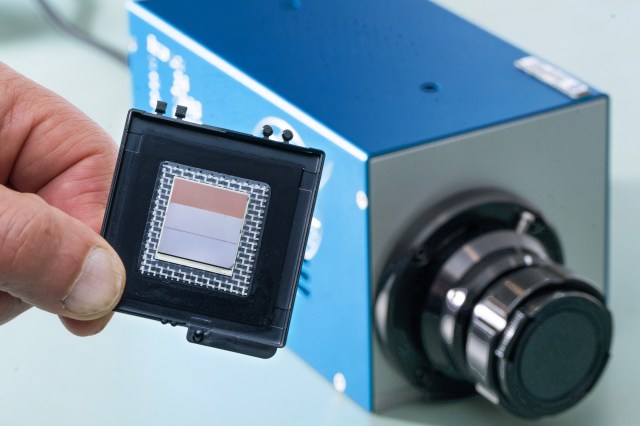
NASA’s Compact Infrared Cameras Enable New Science
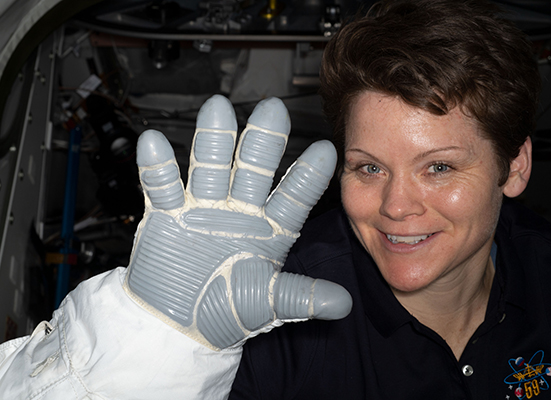
Tech Today: From Spacesuits to Racing Suits
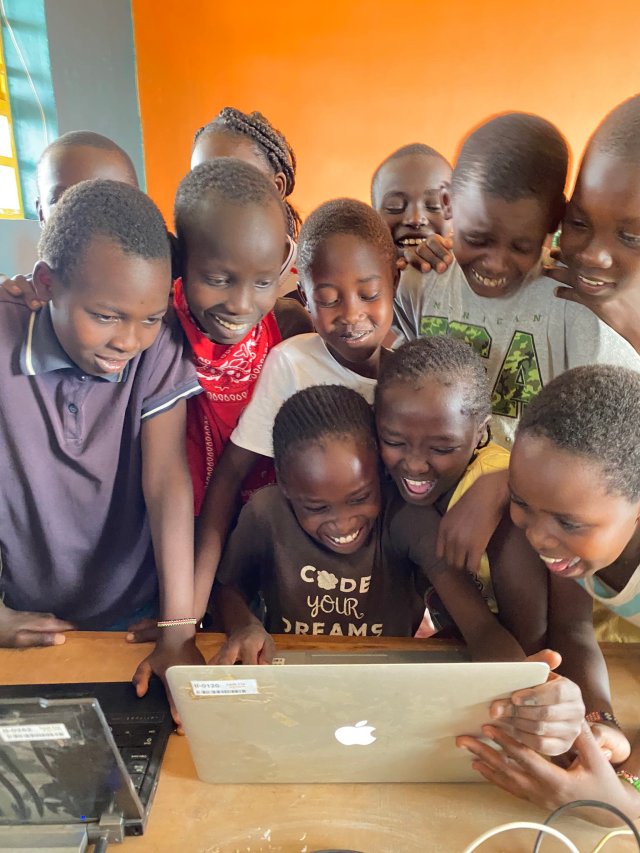
NASA Around the World: Interns Teach Virtual Lessons in Kenya
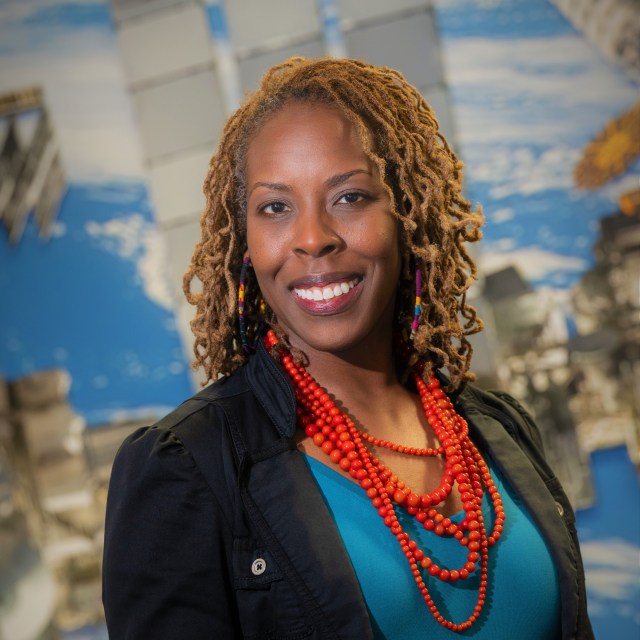
Jennifer Scott Williams: Leading the Next Giant Leap in Space Exploration and Championing STEM Advocacy
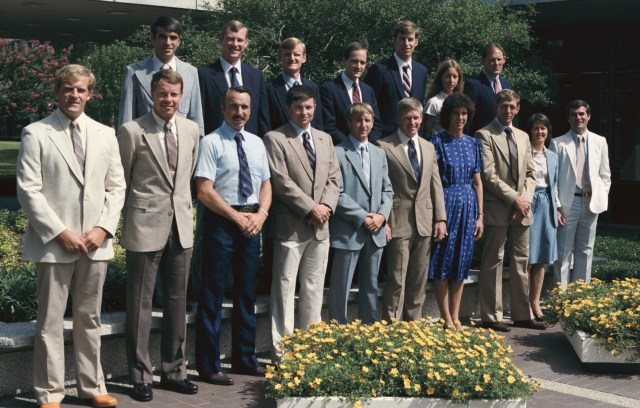
40 Years Ago: NASA Selects its 10th Group of Astronauts
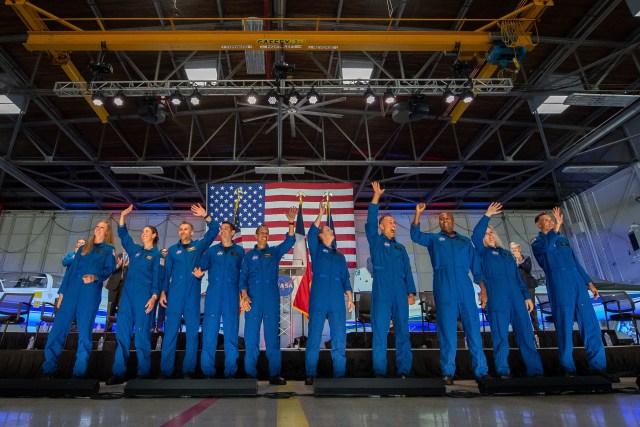
Diez maneras en que los estudiantes pueden prepararse para ser astronautas
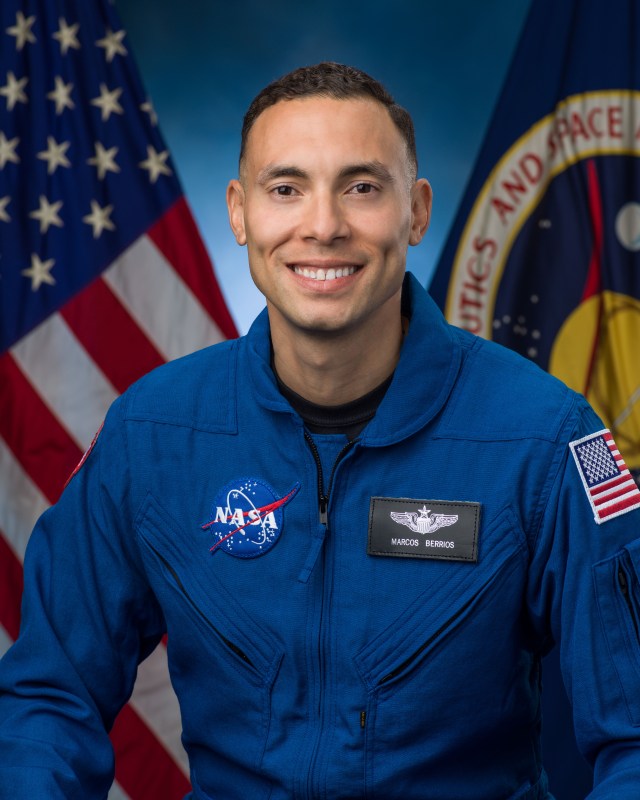
Astronauta de la NASA Marcos Berríos
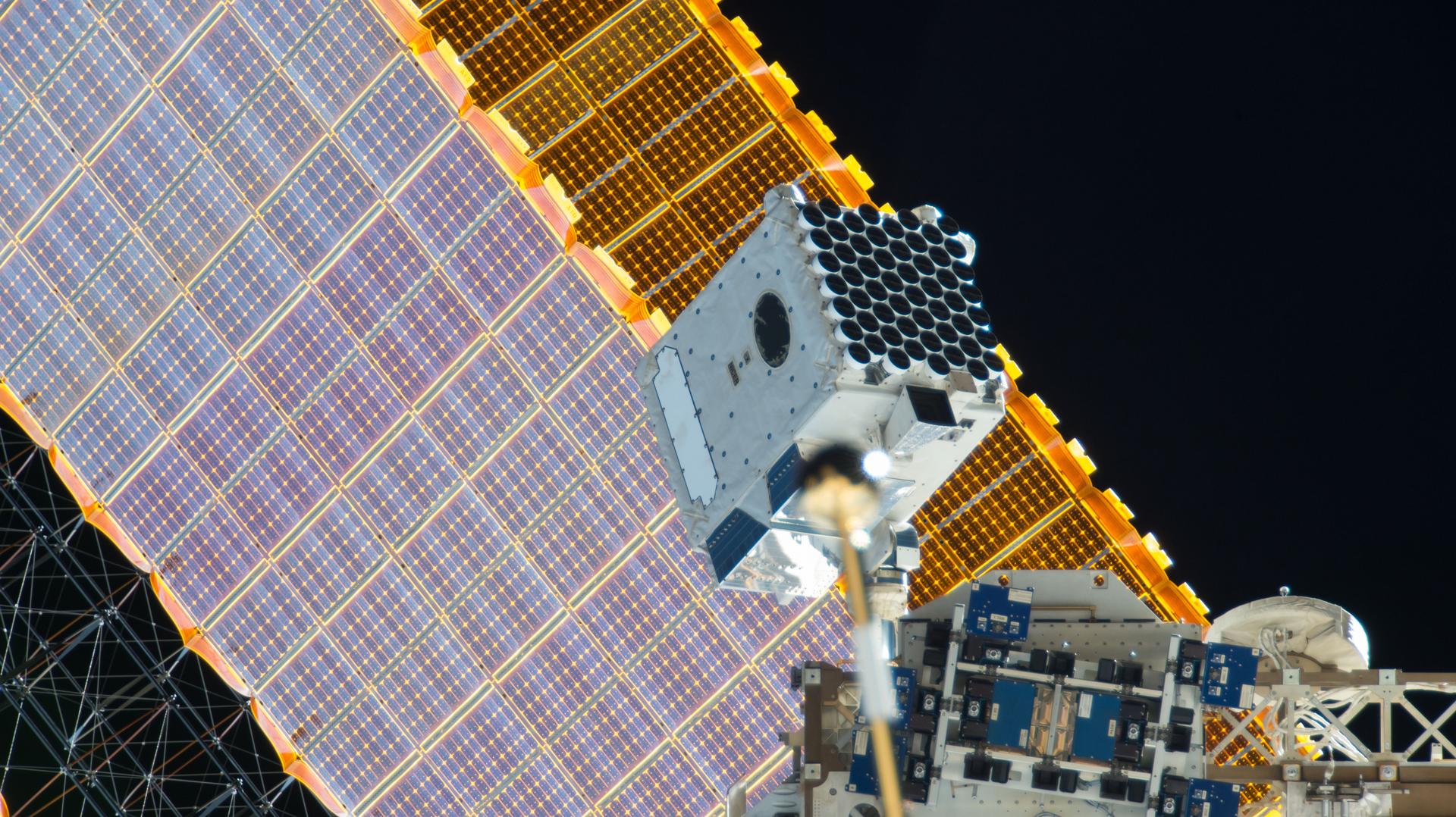
Resultados científicos revolucionarios en la estación espacial de 2023
Key building block for organic molecules discovered in meteorites.
William Steigerwald
Scientists from Japan and NASA have confirmed the presence in meteorites of a key organic molecule which may have been used to build other organic molecules, including some used by life. The discovery validates theories of the formation of organic compounds in extraterrestrial environments.
The chemistry of life runs on organic compounds, molecules containing carbon and hydrogen, which also may include oxygen, nitrogen and other elements. While commonly associated with life, organic molecules also can be created by non-biological processes and are not necessarily indicators of life. An enduring mystery regarding the origin of life is how biology could have arisen from non-biological chemical processes, called prebiotic chemistry. Organic molecules from meteorites may be one of the sources of organic compounds that led to the emergence of life on Earth.
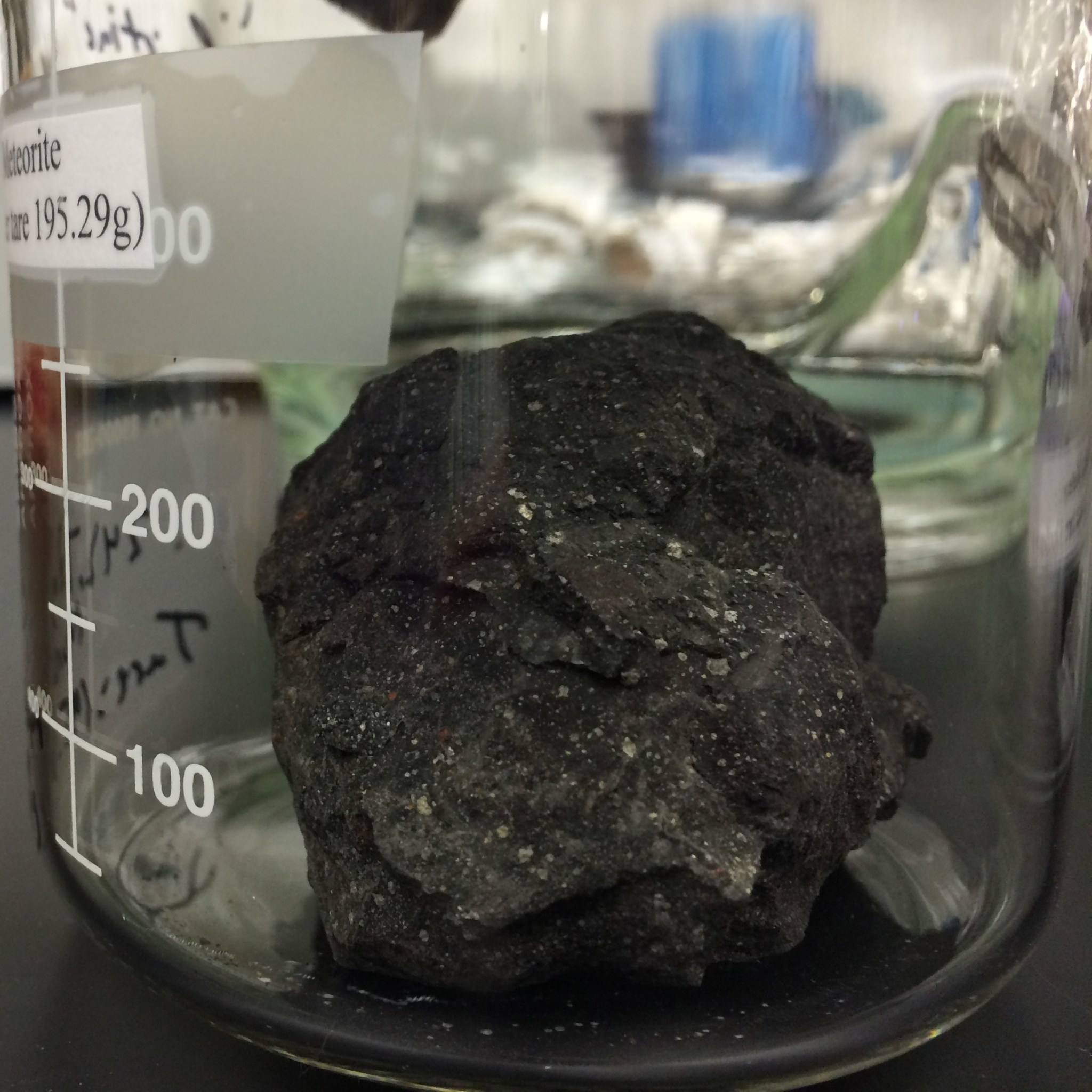
Associate Professor Yasuhiro Oba from Hokkaido University, Japan, led an international team of researchers who discovered the presence of a prebiotic organic molecule called hexamethylenetetramine (HMT) in three different carbon-rich meteorites. Their discovery validates models and theories that propose HMT as an important molecule in the formation of organic compounds in interstellar environments.
“HMT is a key piece of a puzzle which draws the whole picture of chemical evolution in space,” said Oba, lead author of a paper about the research published December 7 in the journal Nature Communications. “To explain the formation of meteoritic organic molecules such as amino acids and sugars, two easily vaporized (volatile) molecules, formaldehyde and ammonia, are necessary in asteroids, the parent bodies of many meteorites. However, since they are easily lost from asteroidal environments due to their high volatility, scientists question how enough could have been available to build the meteoritic organic molecules being found. HMT does not vaporize even at room temperature, and it can produce both molecules if it is heated with liquid water inside asteroids. Finding HMT in meteorites confirms the hypothesis that it is a stable source for ammonia and formaldehyde in asteroids.”
Early in the solar system’s history, many asteroids could have been heated by collisions or the decay of radioactive elements. If some asteroids were warm enough and had liquid water, HMT could have broken down to provide building blocks such as formaldehyde and ammonia that in turn reacted to make other important biological molecules which have been found in meteorites, including amino acids. Some types of amino acids are used by life to make proteins, which are used to build structures like hair and nails, or to speed up or regulate chemical reactions.
“These results shed light on the various ways amino acids can form in extraterrestrial environments,” said Jason Dworkin , a co-author of the paper at NASA’s Goddard Space Flight Center in Greenbelt, Maryland. “This can be explored further when comparing the samples from Japan’s Hayabusa2 and NASA’s OSIRIS-REx missions. These spacecraft collected material from asteroids with what appears to be different histories of liquid water. If there is a mission to return a sample from a comet nucleus someday, perhaps we can see if there is a connection between HMT in comets and asteroids.”
While the diversity of organic compounds in meteorites is well-documented, many questions remain about the processes by which these compounds were formed. The most important meteorites in this area of research are carbonaceous chondrites, stony meteorites that contain high percentages of water and organic compounds. Experimental models have shown that a combination of water, ammonia and methanol, when subjected to photochemical and thermal conditions common in extraterrestrial environments, give rise to a number of organic compounds, the most common of which is HMT. Interstellar ice is rich in methanol. Hypothetically, HMT should be common in water-containing extraterrestrial materials, but, until this study, it had not been detected.
HMT is likely to break apart when exposed to processes commonly used in the analysis of organic compounds in meteorites, and therefore, may not have been detected in other studies even though it was present. The scientists developed a method that specifically extracted HMT from meteorites with minimal breakdown. This method allowed them to isolate significant quantities of HMT and HMT derivatives from the meteorites Murchison, Murray and Tagish Lake.
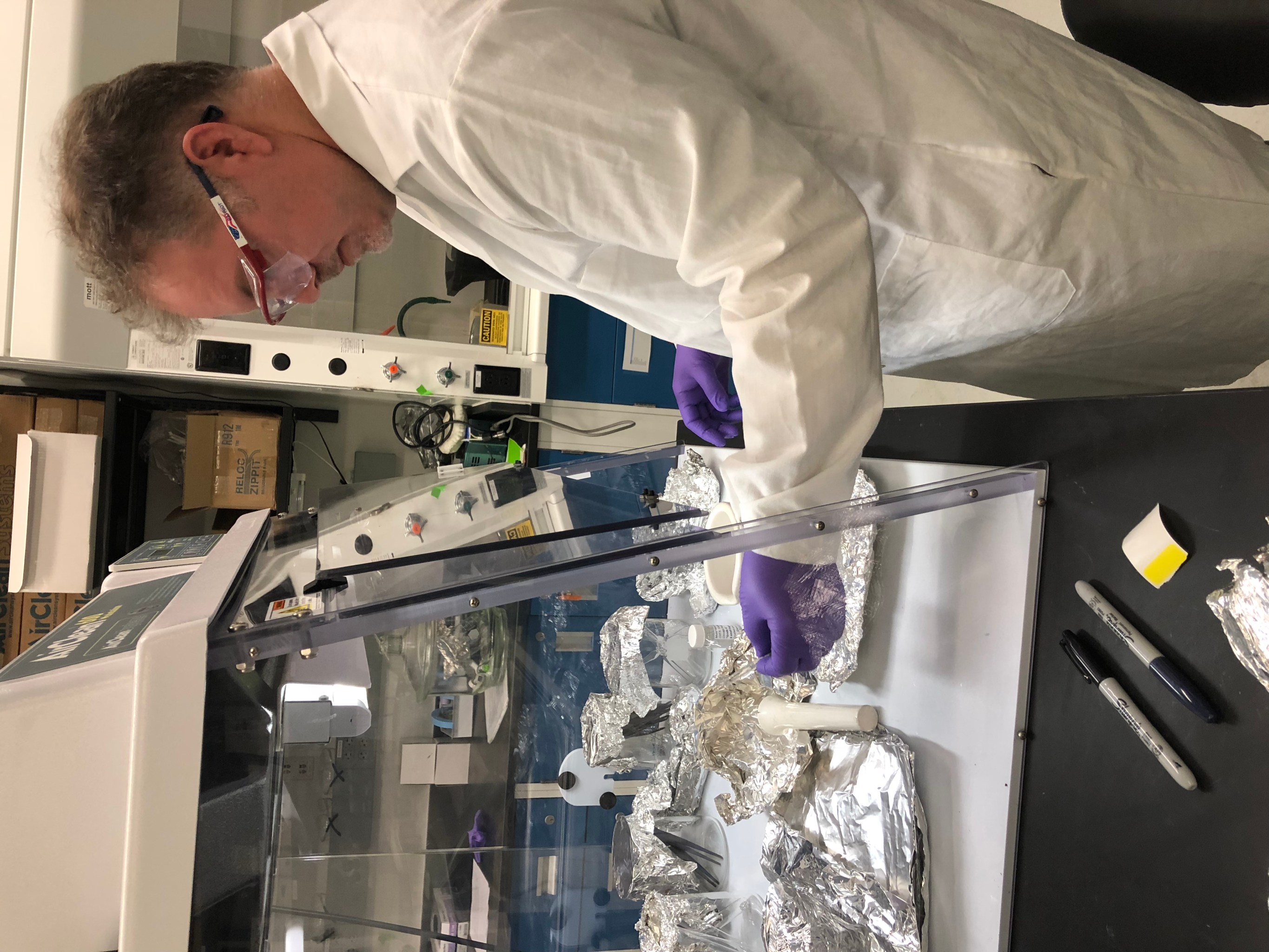
Since Earth has abundant life, the researchers had to be confident that the HMT found in the meteorites was in fact extraterrestrial, and not just from contamination by terrestrial life. “The Murchison fragment used in this study was from the Chicago Field Museum that had been stored for many years inside a sealed container, and is the least contaminated and most pristine piece of Murchison we have ever studied for amino acids, giving us more confidence that the HMT detected in this meteorite is in fact extraterrestrial in origin,” said Daniel Glavin of NASA Goddard, a co-author on the study.
This work was supported by the Japan Society for the Promotion of Science (JSPS) KAKENHI (JP15H05749, JP16H04083, JP17H04862, JP20H00202), the National Aeronautics and Space Administration (NASA) Astrobiology Institute through the Goddard Center for Astrobiology (13-13NAI7-0032), NASA’s Planetary Science Division Internal Scientist Funding Program through the Fundamental Laboratory Research (FLaRe) work package at NASA Goddard Space Flight Center, and the Simons Foundation (SCOL award 302497).
Yasuhiro Oba is part of the Astrophysical Chemistry/Ice & Planetary Science Group at the Institute of Low Temperature Science, where he studies chemical evolution of compounds at scales from molecular clouds to planetary systems.
Bill Steigerwald
NASA Goddard Space Flight Center, Greenbelt, Maryland

- May 26, 2024 | AI Breakthrough: Over a Hundred New Cosmic Absorbers Discovered
- May 25, 2024 | Energy From the Sky: How Drones Can Generate Electricity
- May 25, 2024 | Alarming 500% Surge: Colorectal Cancer Rates Skyrocket Among U.S. Youths
- May 25, 2024 | Webb Telescope’s Breakthrough: First Atmospheric Discovery on a Rocky Super-Earth
- May 25, 2024 | Magnetic Fingerprints: STAR Detects Currents in Deconfined Nuclear Matter
New Clues to Origin of Life on Earth From Meteorite Discovery
By NASA Goddard Space Flight Center November 19, 2019
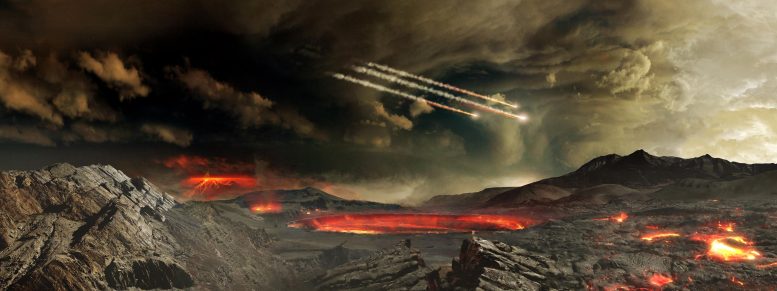
Artist’s concept of meteors impacting ancient Earth. Some scientists think such impacts may have delivered water and other molecules useful to emerging life on Earth. Credit: NASA’s Goddard Space Flight Center Conceptual Image Lab
An international team has found sugars essential to life in meteorites. The new discovery adds to the growing list of biologically important compounds that have been found in meteorites, supporting the hypothesis that chemical reactions in asteroids – the parent bodies of many meteorites – can make some of life’s ingredients. If correct, meteorite bombardment on ancient Earth may have assisted the origin of life with a supply of life’s building blocks.
The team discovered ribose and other bio-essential sugars including arabinose and xylose in two different meteorites that are rich in carbon, NWA 801 (type CR2) and Murchison (type CM2). Ribose is a crucial component of RNA (ribonucleic acid ). In much of modern life, RNA serves as a messenger molecule, copying genetic instructions from the DNA molecule (deoxyribonucleic acid) and delivering them to molecular factories within the cell called ribosomes that read the RNA to build specific proteins needed to carry out life processes.
“Other important building blocks of life have been found in meteorites previously, including amino acids (components of proteins) and nucleobases (components of DNA and RNA), but sugars have been a missing piece among the major building blocks of life,” said Yoshihiro Furukawa of Tohoku University, Japan, lead author of the study published in the Proceedings of the National Academy of Sciences on November 18, 2019. “The research provides the first direct evidence of ribose in space and the delivery of the sugar to Earth. The extraterrestrial sugar might have contributed to the formation of RNA on the prebiotic Earth which possibly led to the origin of life.”
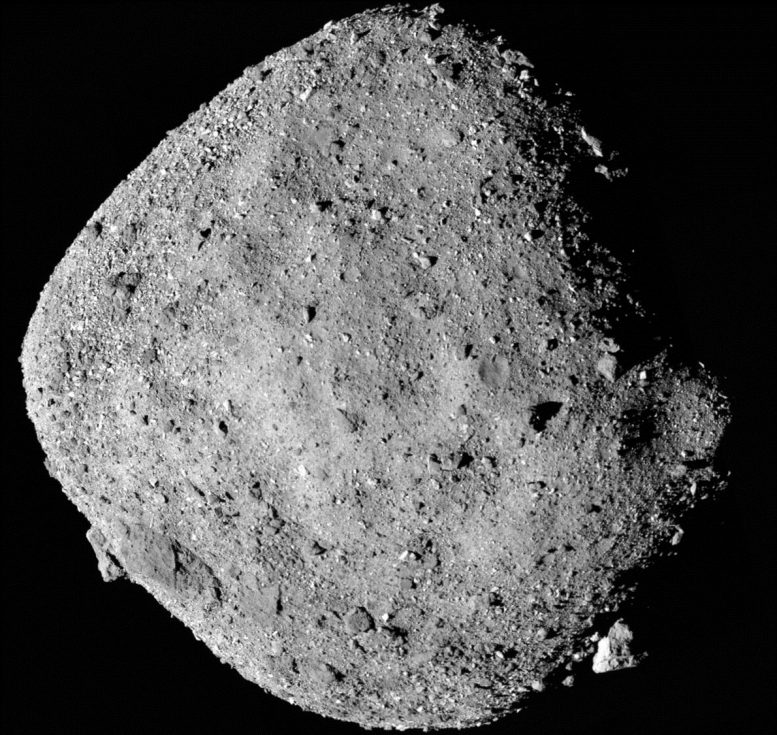
This is a mosaic image of asteroid Bennu, from NASA’s OSIRIS-REx spacecraft. The discovery of sugars in meteorites supports the hypothesis that chemical reactions in asteroids – the parent bodies of many meteorites – can make some of life’s ingredients. Credit: NASA/Goddard/University of Arizona
“It is remarkable that a molecule as fragile as ribose could be detected in such ancient material,” said Jason Dworkin, a co-author of the study at NASA ’s Goddard Space Flight Center in Greenbelt, Maryland. “These results will help guide our analyses of pristine samples from primitive asteroids Ryugu and Bennu, to be returned by the Japan Aerospace Exploration Agency’s Hayabusa2 and NASA’s OSIRIS-REx spacecraft.”
An enduring mystery regarding the origin of life is how biology could have arisen from non-biological chemical processes. DNA is the template for life, carrying the instructions for how to build and operate a living organism. However, RNA also carries information, and many researchers think it evolved first and was later replaced by DNA. This is because RNA molecules have capabilities that DNA lacks. RNA can make copies of itself without “help” from other molecules, and it can also initiate or speed up chemical reactions as a catalyst . The new work gives some evidence to support the possibility that RNA coordinated the machinery of life before DNA.
“The sugar in DNA (2-deoxyribose) was not detected in any of the meteorites analyzed in this study,” said Danny Glavin, a co-author of the study at NASA Goddard. “This is important since there could have been a delivery bias of extraterrestrial ribose to the early Earth which is consistent with the hypothesis that RNA evolved first.”
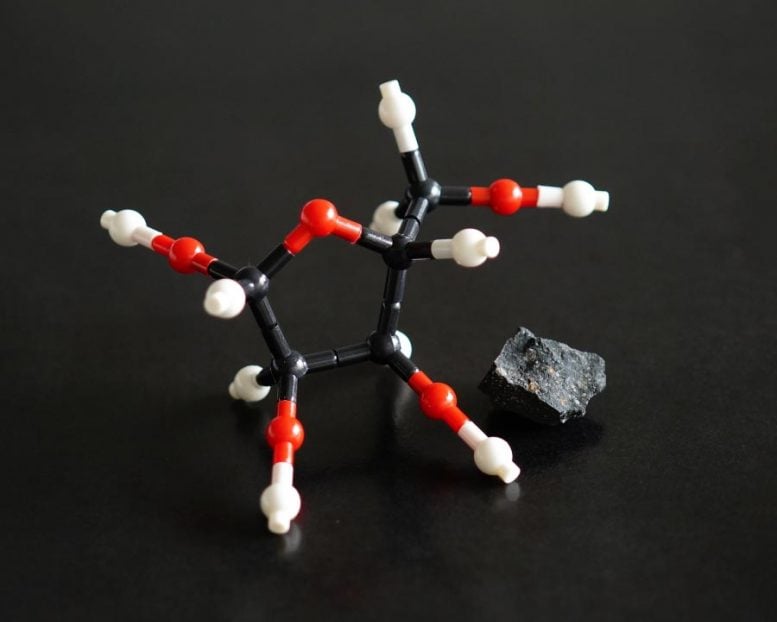
This is a model of the molecular structure of ribose and an image of the Murchison meteorite. Ribose and other sugars were found in this meteorite. Credit: Yoshihiro Furukawa
The team discovered the sugars by analyzing powdered samples of the meteorites using gas chromatography mass spectrometry, which sorts and identifies molecules by their mass and electric charge. They found that the abundances of ribose and the other sugars ranged from 2.3 to 11 parts per billion in NWA 801 and from 6.7 to 180 parts per billion in Murchison.
Since Earth is awash with life, the team had to consider the possibility that the sugars in the meteorites simply came from contamination by terrestrial life. Multiple lines of evidence indicate contamination is unlikely, including isotope analysis. Isotopes are versions of an element with different mass due to the number of neutrons in the atomic nucleus. For example, life on Earth prefers to use the lighter variety of carbon ( 12 C) over the heavier version ( 13 C). However, the carbon in the meteorite sugars was significantly enriched in the heavy 13 C, beyond the amount seen in terrestrial biology, supporting the conclusion that it came from space.
The team plans to analyze more meteorites to get a better idea of the abundance of the extraterrestrial sugars. They also plan to see if the extraterrestrial sugar molecules have a left-handed or right-handed bias. Some molecules come in two varieties that are mirror images of each other, like your hands. On Earth, life uses left-handed amino acids and right-handed sugars. Since it’s possible that the opposite would work fine – right-handed amino acids and left-handed sugars – scientists want to know where this preference came from. If some process in asteroids favors the production of one variety over the other, then maybe the supply from space via meteorite impacts made that variety more abundant on ancient Earth, which made it more likely that life would end up using it.
Read First Evidence of Bio-Essential Sugars in Meteorites for more on this discovery.
Reference: “Extraterrestrial ribose and other sugars in primitive meteorites” by Yoshihiro Furukawa, Yoshito Chikaraishi, Naohiko Ohkouchi, Nanako O. Ogawa, Daniel P. Glavin, Jason P. Dworkin, Chiaki Abe and Tomoki Nakamura, 18 November 2019, Proceedings of the National Academy of Sciences . DOI: 10.1073/pnas.1907169116
The research was funded by a Japan Society for the Promotion of Science KAKENHI (science grant), the National Institutes of Natural Sciences Astrobiology Center, Japan, the Institute of Low Temperature Science, Hokkaido University , the Simons Foundation, and the NASA Astrobiology Institute, Goddard Center for Astrobiology. Jason Dworkin and Danny Glavin are members of the Goddard Center for Astrobiology team.
More on SciTechDaily
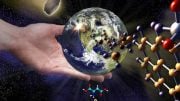
Amino Acids in Meteorites Provide a Clue to How Life Turned Left
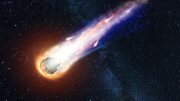
First Evidence of Bio-Essential Sugars in Meteorites
Pristine extraterrestrial organic compounds discovered in “fireball” meteorite.
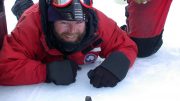
Pristine Space Rock Offers NASA a Peek at the Building Blocks of the Evolution of Life
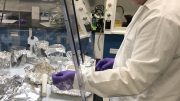
Meteorite Discovery: Key Building Block for Organic Molecules
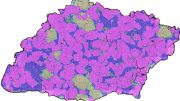
Martian Meteorite Contains Chemical Implicated in the Origin of Life
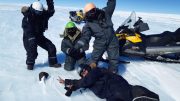
Meteorite Hunters Discover Extraordinary 17-Pound Space Rock in Antarctica
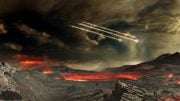
Compounds Discovered in Meteorites May Hold Clues to the Origin of Life
Be the first to comment on "new clues to origin of life on earth from meteorite discovery", leave a comment cancel reply.
Email address is optional. If provided, your email will not be published or shared.
Save my name, email, and website in this browser for the next time I comment.
Meteorites are space rocks that fall to Earth's surface.
Earth Science, Astronomy, Geology, Meteorology, Geography, Physical Geography
Loading ...
Meteorites are space rocks that fall to Earth’s surface. Meteorites are the last stage in the existence of these type of space rocks. Before they were meteorites, the rocks were meteors. Before they were meteors, they were meteoroids . Meteoroids are lumps of rock or metal that orbit the sun . Meteoroids become meteors when they crash into Earth’s atmosphere and the gases surrounding them briefly light up as “shooting stars.” While most meteors burn up and disintegrate in the atmosphere, many of these space rocks reach Earth’s surface in the form of meteorites.
Dust -sized particles called micrometeorites make up 99 percent of the approximately 50 tons of space debris that falls on Earth’s surface every day. Some meteorites, however, are as large as boulders . The largest meteorite found on Earth is the Hoba meteorite discovered in Namibia in 1920. The Hoba meteorite weighs roughly 54,000 kilograms (119,000 pounds). The Hoba meteorite is so big, and so heavy, it has never been moved from where it was found!
Most meteorites look very much like rocks found on Earth, except meteorites usually have a dark, burned exterior . This exterior is formed as friction from the atmosphere melts the meteorite as it crashes toward Earth. Known as thermal ablation , this process can also give meteorites a roughened, smooth, or thumbprint surface.
Thermal ablation creates these different textures due to different chemicals present in the meteorite. Meteorites crash through the atmospheres of all planets and moons in our solar system . Some planets and moons don't have enough atmosphere to break apart meteors, resulting in large meteorites. These larger meteorites create deep, round impact craters that can be found all over our Moon, Mercury, and Mars . In 2005, the first meteorite found on another planet was discovered by Opportunity, one of NASA’s Mars rover spacecraft . In 2014, Opportunity ’s sister spacecraft, Curiosity, discovered a meteorite that was two meters (seven feet) wide, making it the largest yet discovered on Mars.
Types of Meteorites
More than 60,000 meteorites have been found on Earth. Scientists have divided these meteorites into three main types: stony, iron, and stony-iron. Each of these types has many sub-groups.
Stony Meteorites
Stony meteorites are made up of minerals that contain silicates —material made of silicon and oxygen. They also contain some metal— nickel and iron. There are two major types of stony meteorites: chondrites and achondrites .
Chondrites themselves are classified into two major groups: ordinary and carbonaceous. Ordinary chondrites are the most common type of stony meteorite, accounting for 86 percent of all meteorites that have fallen to Earth. They are named for the hardened droplets of lava , called chondrules , embedded in them. Chondrites formed from the dust and small particles that came together to form asteroids in the early solar system, more than 4.5 billion years ago. Because they were formed at the same time as the solar system, chondrites are integral to the study of the solar system’s origin, age, and composition .
Ordinary chondrites can be classified into three main groups. The groups indicate the meteorite’s quantity of iron. The H chondrite group has a h igh amount of iron. The L chondrite group has a l ow amount of iron. The LL group has a l ow amount of iron and a l ow amount of metal in general. Carbonaceous chondrites are much more rare than ordinary chondrites. Astronomers think carbonaceous chondrites formed far away from the sun as the early solar system developed. As their name implies, carbonaceous chondrites contain the element carbon, usually in the form of organic compounds such as amino acids .
Carbonaceous chondrites also often contain water or material that was shaped by the presence of water. Like ordinary chondrites, carbonaceous chondrites can be more minutely classified based on their mineral composition. All groups of carbonaceous chondrites are marked with a two- or three-letter code starting with C. Carbonaceous chondrites are often named after the first specimen of that type recovered. The CI group, for instance, is named after the Ivuna meteorite, which crashed into Tanzania in 1938. CI meteorites have a high amount of carbon, as well as clays .
Carbonaceous chondrites can also be named after the place where the first specimen of the type was found. The CV group is named after a meteorite that crashed near the city of Vigarano, Italy, in 1910. The most famous CV meteorite is probably the Allende meteorite, which fell to Earth near Pueblo de Allende, Chihuahua, Mexico, in 1969. The Allende meteorite has thousands of tiny chondrules made of the mineral olivine . The Allende meteorite also has grains of a special kind of carbon: diamonds . These diamonds are actually older than the solar system, and astronomers think they were produced as blast material from a nearby, ancient supernova .
Achondrites do not contain the lava droplets (chondrules) present in chondrites. They are very rare, making up about 3 percent of all known meteorites. Most achondrites form from the brittle outer layers of asteroids, which are similar to Earth’s crust . There are many classifications of achondrites. The “ primitive achondrite” group, for instance, has a very similar mineral composition to chondrites. Lunar meteorites are achondrites that crashed to Earth from the moon, while Martian achondrites crashed to Earth from our neighbor planet, Mars. Very few meteorites, only about 0.2 percent, come from Mars and the moon. These achondrites are the results of Mars and the moon’s own meteorite impacts . Large meteorites hit the surface of Mars and the moon, blasting off bits of rock. These rock bits rarely make their way to our atmosphere as meteors and even more rarely hit Earth’s surface.
Iron Meteorites
Iron meteorites are mostly made of iron and nickel. They come from the cores of asteroids and account for about 5 percent of meteorites on Earth. Iron meteorites are the most massive meteorites ever discovered. Their heavy mineral composition (iron and nickel) often allows them to survive the harsh plummet through Earth’s atmosphere without breaking into smaller pieces. The largest meteorite ever found, Namibia’s Hoba meteorite, is an iron meteorite.
Stony-Iron Meteorites
Stony-iron meteorites have nearly equal amounts of silicate minerals (chemicals that contain the elements silicon and oxygen) and metals (iron and nickel). One group of stony-iron meteorites, the pallasites , contains yellow-green olivine crystals encased in shiny metal. Astronomers think many pallasites are relics of an asteroid’s core- mantle boundary . Their chemical composition is similar to many iron meteorites, leading astronomers to think maybe they came from different parts of the same asteroid that broke up when it crashed into Earth’s atmosphere.
Meteorite Impact Craters
Meteorites crash through Earth’s atmosphere with tremendous force. The largest meteorites leave enormous holes in the ground called impact craters. The best-preserved impact crater in the world is the Barringer Meteorite Crater, near the U.S. town of Winslow, Arizona. There, more than 50,000 years ago, a meteorite weighing about 270,000 metric tons (300,000 tons) slammed into Earth with the force of 2.5 million tons of TNT. The impact blasted a hole one kilometer (0.6 miles) wide and about 230 meters (750 feet) deep. The fragments left of the space rock show that it was an iron meteorite. More than a hundred impact craters have been identified on Earth. Perhaps the most famous is the Chicxulub Crater, in Yucatan, Mexico. The Chicxulub Crater can be identified on land, beneath dozens of meters of sediment , although about half of the feature is submerged in the Gulf of Mexico. It is one of the largest impact craters ever discovered on Earth. Despite its size, the Chicxulub Crater is famous for another reason. Many scientists think the large meteorite that created the Chicxulub Crater—measuring roughly 10 kilometers (six miles) wide— triggered the extinction of the dinosaurs and other animal and plant life 66 million years ago.
Ablation Blackening
Thermal ablation, the process that burns off the surface layer of a meteorite and causes it to appear blackened, is the same process that blackens the outside of returning spacecraft, such as tiles on the space shuttle.
Mars and the Moon
As of July 2014, there were 133 Martian meteorites and 183 lunar meteorites found on Earth—not a lot. Scientists are able to tell the rocks came from Mars and the Moon because their respective compositions matches chemical analysis of rocks conducted during NASA’s robotic explorations of Mars and the “moon rocks” recovered during the Apollo lunar missions.
Meteorite or Meteorwrong? How can you tell if that rock you found fell from the sky? First of all, meteorites get burned when they enter Earth's atmosphere, so they are usually black and crusty on the outside. Also, meteorites, even stony meteorites, contain iron, so a magnet will stick to them.
Natural Hazards
Most meteorites fall to Earth harmlessly. Sometimes, however, they can cause great damage. The extinction of most life on Earth 66 million years ago is a good example of that. A less catastrophic impact hit a driveway in the U.S. town of Peekskill, New York, in 1992. Although no one was harmed, the meteorite slammed through the trunk of a parked Chevrolet Malibu, barely missing the gas tank, before creating a small impact crater beneath the car. The Chevy is nicknamed the "Peekskill Meteorite Car."
Rocky Cookie The best place to hunt for meteorites is in Antarctica. Because most of Antarctica is covered in ice and snow, rocky meteorites stand out like chocolate chips in a cookie.
Media Credits
The audio, illustrations, photos, and videos are credited beneath the media asset, except for promotional images, which generally link to another page that contains the media credit. The Rights Holder for media is the person or group credited.
Last Updated
April 4, 2024
User Permissions
For information on user permissions, please read our Terms of Service. If you have questions about how to cite anything on our website in your project or classroom presentation, please contact your teacher. They will best know the preferred format. When you reach out to them, you will need the page title, URL, and the date you accessed the resource.
If a media asset is downloadable, a download button appears in the corner of the media viewer. If no button appears, you cannot download or save the media.
Text on this page is printable and can be used according to our Terms of Service .
Interactives
Any interactives on this page can only be played while you are visiting our website. You cannot download interactives.
Related Resources
1.1 The Science of Biology
Learning objectives.
By the end of this section, you will be able to do the following:
- Identify the shared characteristics of the natural sciences
- Summarize the steps of the scientific method
- Compare inductive reasoning with deductive reasoning
- Describe the goals of basic science and applied science
What is biology? In simple terms, biology is the study of life. This is a very broad definition because the scope of biology is vast. Biologists may study anything from the microscopic or submicroscopic view of a cell to ecosystems and the whole living planet ( Figure 1.2 ). Listening to the daily news, you will quickly realize how many aspects of biology we discuss every day. For example, recent news topics include Escherichia coli ( Figure 1.3 ) outbreaks in spinach and Salmonella contamination in peanut butter. Other subjects include efforts toward finding a cure for AIDS, Alzheimer’s disease, and cancer. On a global scale, many researchers are committed to finding ways to protect the planet, solve environmental issues, and reduce the effects of climate change. All of these diverse endeavors are related to different facets of the discipline of biology.
The Process of Science
Biology is a science, but what exactly is science? What does the study of biology share with other scientific disciplines? We can define science (from the Latin scientia , meaning “knowledge”) as knowledge that covers general truths or the operation of general laws, especially when acquired and tested by the scientific method. It becomes clear from this definition that applying scientific method plays a major role in science. The scientific method is a method of research with defined steps that include experiments and careful observation.
We will examine scientific method steps in detail later, but one of the most important aspects of this method is the testing of hypotheses by means of repeatable experiments. A hypothesis is a suggested explanation for an event, which one can test. Although using the scientific method is inherent to science, it is inadequate in determining what science is. This is because it is relatively easy to apply the scientific method to disciplines such as physics and chemistry, but when it comes to disciplines like archaeology, psychology, and geology, the scientific method becomes less applicable as repeating experiments becomes more difficult.
These areas of study are still sciences, however. Consider archaeology—even though one cannot perform repeatable experiments, hypotheses may still be supported. For instance, archaeologists can hypothesize that an ancient culture existed based on finding a piece of pottery. They could make further hypotheses about various characteristics of this culture, which could be correct or false through continued support or contradictions from other findings. A hypothesis may become a verified theory. A theory is a tested and confirmed explanation for observations or phenomena. Therefore, we may be better off to define science as fields of study that attempt to comprehend the nature of the universe.
Natural Sciences
What would you expect to see in a museum of natural sciences? Frogs? Plants? Dinosaur skeletons? Exhibits about how the brain functions? A planetarium? Gems and minerals? Maybe all of the above? Science includes such diverse fields as astronomy, biology, computer sciences, geology, logic, physics, chemistry, and mathematics ( Figure 1.4 ). However, scientists consider those fields of science related to the physical world and its phenomena and processes natural sciences . Thus, a museum of natural sciences might contain any of the items listed above.
There is no complete agreement when it comes to defining what the natural sciences include, however. For some experts, the natural sciences are astronomy, biology, chemistry, earth science, and physics. Other scholars choose to divide natural sciences into life sciences , which study living things and include biology, and physical sciences , which study nonliving matter and include astronomy, geology, physics, and chemistry. Some disciplines such as biophysics and biochemistry build on both life and physical sciences and are interdisciplinary. Some refer to natural sciences as “hard science” because they rely on the use of quantitative data. Social sciences that study society and human behavior are more likely to use qualitative assessments to drive investigations and findings.
Not surprisingly, the natural science of biology has many branches or subdisciplines. Cell biologists study cell structure and function, while biologists who study anatomy investigate the structure of an entire organism. Those biologists studying physiology, however, focus on the internal functioning of an organism. Some areas of biology focus on only particular types of living things. For example, botanists explore plants, while zoologists specialize in animals.
Scientific Reasoning
One thing is common to all forms of science: an ultimate goal “to know.” Curiosity and inquiry are the driving forces for the development of science. Scientists seek to understand the world and the way it operates. To do this, they use two methods of logical thinking: inductive reasoning and deductive reasoning.
Inductive reasoning is a form of logical thinking that uses related observations to arrive at a general conclusion. This type of reasoning is common in descriptive science. A life scientist such as a biologist makes observations and records them. These data can be qualitative or quantitative, and one can supplement the raw data with drawings, pictures, photos, or videos. From many observations, the scientist can infer conclusions (inductions) based on evidence. Inductive reasoning involves formulating generalizations inferred from careful observation and analyzing a large amount of data. Brain studies provide an example. In this type of research, scientists observe many live brains while people are engaged in a specific activity, such as viewing images of food. The scientist then predicts the part of the brain that “lights up” during this activity to be the part controlling the response to the selected stimulus, in this case, images of food. Excess absorption of radioactive sugar derivatives by active areas of the brain causes the various areas to "light up". Scientists use a scanner to observe the resultant increase in radioactivity. Then, researchers can stimulate that part of the brain to see if similar responses result.
Deductive reasoning or deduction is the type of logic used in hypothesis-based science. In deductive reasoning, the pattern of thinking moves in the opposite direction as compared to inductive reasoning. Deductive reasoning is a form of logical thinking that uses a general principle or law to predict specific results. From those general principles, a scientist can deduce and predict the specific results that would be valid as long as the general principles are valid. Studies in climate change can illustrate this type of reasoning. For example, scientists may predict that if the climate becomes warmer in a particular region, then the distribution of plants and animals should change.
Both types of logical thinking are related to the two main pathways of scientific study: descriptive science and hypothesis-based science. Descriptive (or discovery) science , which is usually inductive, aims to observe, explore, and discover, while hypothesis-based science , which is usually deductive, begins with a specific question or problem and a potential answer or solution that one can test. The boundary between these two forms of study is often blurred, and most scientific endeavors combine both approaches. The fuzzy boundary becomes apparent when thinking about how easily observation can lead to specific questions. For example, a gentleman in the 1940s observed that the burr seeds that stuck to his clothes and his dog’s fur had a tiny hook structure. On closer inspection, he discovered that the burrs’ gripping device was more reliable than a zipper. He eventually experimented to find the best material that acted similarly, and produced the hook-and-loop fastener popularly known today as Velcro. Descriptive science and hypothesis-based science are in continuous dialogue.
The Scientific Method
Biologists study the living world by posing questions about it and seeking science-based responses. Known as scientific method, this approach is common to other sciences as well. The scientific method was used even in ancient times, but England’s Sir Francis Bacon (1561–1626) first documented it ( Figure 1.5 ). He set up inductive methods for scientific inquiry. The scientific method is not used only by biologists; researchers from almost all fields of study can apply it as a logical, rational problem-solving method.
The scientific process typically starts with an observation (often a problem to solve) that leads to a question. Let’s think about a simple problem that starts with an observation and apply the scientific method to solve the problem. One Monday morning, a student arrives at class and quickly discovers that the classroom is too warm. That is an observation that also describes a problem: the classroom is too warm. The student then asks a question: “Why is the classroom so warm?”
Proposing a Hypothesis
Recall that a hypothesis is a suggested explanation that one can test. To solve a problem, one can propose several hypotheses. For example, one hypothesis might be, “The classroom is warm because no one turned on the air conditioning.” However, there could be other responses to the question, and therefore one may propose other hypotheses. A second hypothesis might be, “The classroom is warm because there is a power failure, and so the air conditioning doesn’t work.”
Once one has selected a hypothesis, the student can make a prediction. A prediction is similar to a hypothesis but it typically has the format “If . . . then . . . .” For example, the prediction for the first hypothesis might be, “ If the student turns on the air conditioning, then the classroom will no longer be too warm.”
Testing a Hypothesis
A valid hypothesis must be testable. It should also be falsifiable , meaning that experimental results can disprove it. Importantly, science does not claim to “prove” anything because scientific understandings are always subject to modification with further information. This step—openness to disproving ideas—is what distinguishes sciences from non-sciences. The presence of the supernatural, for instance, is neither testable nor falsifiable. To test a hypothesis, a researcher will conduct one or more experiments designed to eliminate one or more of the hypotheses. Each experiment will have one or more variables and one or more controls. A variable is any part of the experiment that can vary or change during the experiment. The control group contains every feature of the experimental group except it is not given the manipulation that the researcher hypothesizes. Therefore, if the experimental group's results differ from the control group, the difference must be due to the hypothesized manipulation, rather than some outside factor. Look for the variables and controls in the examples that follow. To test the first hypothesis, the student would find out if the air conditioning is on. If the air conditioning is turned on but does not work, there should be another reason, and the student should reject this hypothesis. To test the second hypothesis, the student could check if the lights in the classroom are functional. If so, there is no power failure and the student should reject this hypothesis. The students should test each hypothesis by carrying out appropriate experiments. Be aware that rejecting one hypothesis does not determine whether or not one can accept the other hypotheses. It simply eliminates one hypothesis that is not valid ( Figure 1.6 ). Using the scientific method, the student rejects the hypotheses that are inconsistent with experimental data.
While this “warm classroom” example is based on observational results, other hypotheses and experiments might have clearer controls. For instance, a student might attend class on Monday and realize she had difficulty concentrating on the lecture. One observation to explain this occurrence might be, “When I eat breakfast before class, I am better able to pay attention.” The student could then design an experiment with a control to test this hypothesis.
In hypothesis-based science, researchers predict specific results from a general premise. We call this type of reasoning deductive reasoning: deduction proceeds from the general to the particular. However, the reverse of the process is also possible: sometimes, scientists reach a general conclusion from a number of specific observations. We call this type of reasoning inductive reasoning, and it proceeds from the particular to the general. Researchers often use inductive and deductive reasoning in tandem to advance scientific knowledge ( Figure 1.7 ). In recent years a new approach of testing hypotheses has developed as a result of an exponential growth of data deposited in various databases. Using computer algorithms and statistical analyses of data in databases, a new field of so-called "data research" (also referred to as "in silico" research) provides new methods of data analyses and their interpretation. This will increase the demand for specialists in both biology and computer science, a promising career opportunity.
Visual Connection
In the example below, the scientific method is used to solve an everyday problem. Match the scientific method steps (numbered items) with the process of solving the everyday problem (lettered items). Based on the results of the experiment, is the hypothesis correct? If it is incorrect, propose some alternative hypotheses.
Decide if each of the following is an example of inductive or deductive reasoning.
- All flying birds and insects have wings. Birds and insects flap their wings as they move through the air. Therefore, wings enable flight.
- Insects generally survive mild winters better than harsh ones. Therefore, insect pests will become more problematic if global temperatures increase.
- Chromosomes, the carriers of DNA, are distributed evenly between the daughter cells during cell division. Therefore, each daughter cell will have the same chromosome set as the mother cell.
- Animals as diverse as humans, insects, and wolves all exhibit social behavior. Therefore, social behavior must have an evolutionary advantage.
The scientific method may seem too rigid and structured. It is important to keep in mind that, although scientists often follow this sequence, there is flexibility. Sometimes an experiment leads to conclusions that favor a change in approach. Often, an experiment brings entirely new scientific questions to the puzzle. Many times, science does not operate in a linear fashion. Instead, scientists continually draw inferences and make generalizations, finding patterns as their research proceeds. Scientific reasoning is more complex than the scientific method alone suggests. Notice, too, that we can apply the scientific method to solving problems that aren’t necessarily scientific in nature.
Two Types of Science: Basic Science and Applied Science
The scientific community has been debating for the last few decades about the value of different types of science. Is it valuable to pursue science for the sake of simply gaining knowledge, or does scientific knowledge only have worth if we can apply it to solving a specific problem or to bettering our lives? This question focuses on the differences between two types of science: basic science and applied science.
Basic science or “pure” science seeks to expand knowledge regardless of the short-term application of that knowledge. It is not focused on developing a product or a service of immediate public or commercial value. The immediate goal of basic science is knowledge for knowledge’s sake, although this does not mean that, in the end, it may not result in a practical application.
In contrast, applied science or “technology,” aims to use science to solve real-world problems, making it possible, for example, to improve a crop yield, find a cure for a particular disease, or save animals threatened by a natural disaster ( Figure 1.8 ). In applied science, the problem is usually defined for the researcher.
Some individuals may perceive applied science as “useful” and basic science as “useless.” A question these people might pose to a scientist advocating knowledge acquisition would be, “What for?” However, a careful look at the history of science reveals that basic knowledge has resulted in many remarkable applications of great value. Many scientists think that a basic understanding of science is necessary before researchers develop an application, therefore, applied science relies on the results that researchers generate through basic science. Other scientists think that it is time to move on from basic science in order to find solutions to actual problems. Both approaches are valid. It is true that there are problems that demand immediate attention; however, scientists would find few solutions without the help of the wide knowledge foundation that basic science generates.
One example of how basic and applied science can work together to solve practical problems occurred after the discovery of DNA structure led to an understanding of the molecular mechanisms governing DNA replication. DNA strands, unique in every human, are in our cells, where they provide the instructions necessary for life. When DNA replicates, it produces new copies of itself, shortly before a cell divides. Understanding DNA replication mechanisms enabled scientists to develop laboratory techniques that researchers now use to identify genetic diseases, pinpoint individuals who were at a crime scene, and determine paternity. Without basic science, it is unlikely that applied science could exist.
Another example of the link between basic and applied research is the Human Genome Project, a study in which researchers analyzed and mapped each human chromosome to determine the precise sequence of DNA subunits and each gene's exact location. (The gene is the basic unit of heredity represented by a specific DNA segment that codes for a functional molecule. An individual’s complete collection of genes is their genome.) Researchers have studied other less complex organisms as part of this project in order to gain a better understanding of human chromosomes. The Human Genome Project ( Figure 1.9 ) relied on basic research with simple organisms and, later, with the human genome. An important end goal eventually became using the data for applied research, seeking cures and early diagnoses for genetically related diseases.
While scientists usually carefully plan research efforts in both basic science and applied science, note that some discoveries are made by serendipity , that is, by means of a fortunate accident or a lucky surprise. Scottish biologist Alexander Fleming discovered penicillin when he accidentally left a petri dish of Staphylococcus bacteria open. An unwanted mold grew on the dish, killing the bacteria. Fleming's curiosity to investigate the reason behind the bacterial death, followed by his experiments, led to the discovery of the antibiotic penicillin, which is produced by the fungus Penicillium . Even in the highly organized world of science, luck—when combined with an observant, curious mind—can lead to unexpected breakthroughs.
Reporting Scientific Work
Whether scientific research is basic science or applied science, scientists must share their findings in order for other researchers to expand and build upon their discoveries. Collaboration with other scientists—when planning, conducting, and analyzing results—is important for scientific research. For this reason, important aspects of a scientist’s work are communicating with peers and disseminating results to peers. Scientists can share results by presenting them at a scientific meeting or conference, but this approach can reach only the select few who are present. Instead, most scientists present their results in peer-reviewed manuscripts that are published in scientific journals. Peer-reviewed manuscripts are scientific papers that a scientist’s colleagues or peers review. These colleagues are qualified individuals, often experts in the same research area, who judge whether or not the scientist’s work is suitable for publication. The process of peer review helps to ensure that the research in a scientific paper or grant proposal is original, significant, logical, and thorough. Grant proposals, which are requests for research funding, are also subject to peer review. Scientists publish their work so other scientists can reproduce their experiments under similar or different conditions to expand on the findings.
A scientific paper is very different from creative writing. Although creativity is required to design experiments, there are fixed guidelines when it comes to presenting scientific results. First, scientific writing must be brief, concise, and accurate. A scientific paper needs to be succinct but detailed enough to allow peers to reproduce the experiments.
The scientific paper consists of several specific sections—introduction, materials and methods, results, and discussion. This structure is sometimes called the “IMRaD” format. There are usually acknowledgment and reference sections as well as an abstract (a concise summary) at the beginning of the paper. There might be additional sections depending on the type of paper and the journal where it will be published. For example, some review papers require an outline.
The introduction starts with brief, but broad, background information about what is known in the field. A good introduction also gives the rationale of the work. It justifies the work carried out and also briefly mentions the end of the paper, where the researcher will present the hypothesis or research question driving the research. The introduction refers to the published scientific work of others and therefore requires citations following the style of the journal. Using the work or ideas of others without proper citation is plagiarism .
The materials and methods section includes a complete and accurate description of the substances the researchers use, and the method and techniques they use to gather data. The description should be thorough enough to allow another researcher to repeat the experiment and obtain similar results, but it does not have to be verbose. This section will also include information on how the researchers made measurements and the types of calculations and statistical analyses they used to examine raw data. Although the materials and methods section gives an accurate description of the experiments, it does not discuss them.
Some journals require a results section followed by a discussion section, but it is more common to combine both. If the journal does not allow combining both sections, the results section simply narrates the findings without any further interpretation. The researchers present results with tables or graphs, but they do not present duplicate information. In the discussion section, the researchers will interpret the results, describe how variables may be related, and attempt to explain the observations. It is indispensable to conduct an extensive literature search to put the results in the context of previously published scientific research. Therefore, researchers include proper citations in this section as well.
Finally, the conclusion section summarizes the importance of the experimental findings. While the scientific paper almost certainly answers one or more scientific questions that the researchers stated, any good research should lead to more questions. Therefore, a well-done scientific paper allows the researchers and others to continue and expand on the findings.
Review articles do not follow the IMRAD format because they do not present original scientific findings, or primary literature. Instead, they summarize and comment on findings that were published as primary literature and typically include extensive reference sections.
Scientific Ethics
Scientists must ensure that their efforts do not cause undue damage to humans, animals, or the environment. They also must ensure that their research and communications are free of bias and that they properly balance financial, legal, safety, replicability, and other considerations. All scientists -- and many people in other fields -- have these ethical obligations, but those in the life sciences have a particular obligation because their research may involve people or other living things. Bioethics is thus an important and continually evolving field, in which researchers collaborate with other thinkers and organizations. They work to define guidelines for current practice, and also continually consider new developments and emerging technologies in order to form answers for the years and decades to come.
For example, bioethicists may examine the implications of gene editing technologies, including the ability to create organisms that may displace others in the environment, as well as the ability to “design” human beings. In that effort, ethicists will likely seek to balance the positive outcomes -- such as improved therapies or prevention of certain illnesses -- with negative outcomes.
Unfortunately, the emergence of bioethics as a field came after a number of clearly unethical practices, where biologists did not treat research subjects with dignity and in some cases did them harm. In the 1932 Tuskegee syphilis study, 399 African American men were diagnosed with syphilis but were never informed that they had the disease, leaving them to live with and pass on the illness to others. Doctors even withheld proven medications because the goal of the study was to understand the impact of untreated syphilis on Black men.
While the decisions made in the Tuskegee study are unjustifiable, some decisions are genuinely difficult to make. Bioethicists work to establish moral and dignifying approaches before such decisions come to pass. For example, doctors rely on artificial intelligence and robotics for medical diagnosis and treatment; in the near future, even more responsibility will lie with machines. Who will be responsible for medical decisions? Who will explain to families if a procedure doesn’t go as planned? And, since such treatments will likely be expensive, who will decide who has access to them and who does not? These are all questions bioethicists seek to answer, and are the types of considerations that all scientific researchers take into account when designing and conducting studies.
Bioethics are not simple, and often leave scientists balancing benefits with harm. In this text and course, you will discuss medical discoveries, vaccines, and research that, at their core, have an ethical complexity or, in the view of many, an ethical lapse. In 1951, Henrietta Lacks , a 30-year-old African American woman, was diagnosed with cervical cancer at Johns Hopkins Hospital. Unique characteristics of her illnesses gave her cells the ability to divide continuously, essentially making them “immortal.” Without her knowledge or permission, researchers took samples of her cells and with them created the immortal HeLa cell line. These cells have contributed to major medical discoveries, including the polio vaccine. Many researchers mentioned in subsequent sections of the text relied on HeLa cell research as at least a component of their work related to cancer, AIDS, cell aging, and even very recently in COVID-19 research.
Today, harvesting tissue or organs from a dying patient without consent is not only considered unethical but illegal, regardless of whether such an act could save other patients’ lives. Is it ethical, then, for scientists to continue to use Lacks’s tissues for research, even though they were obtained illegally by today’s standards? Should Lacks be mentioned as a contributor to the research based on her cells, and should she be cited in the several Nobel Prizes that have been awarded through such work? Finally, should medical companies be obligated to pay Lacks’ family (which had financial difficulties) a portion of the billions of dollars in revenue earned through medicines that benefited from HeLa cell research? How would Henrietta Lacks feel about this? Because she was never asked, we will never know.
To avoid such situations, the role of ethics in scientific research is to ask such questions before, during, and after research or practice takes place, as well as to adhere to established professional principles and consider the dignity and safety of all organisms involved or affected by the work.
As an Amazon Associate we earn from qualifying purchases.
This book may not be used in the training of large language models or otherwise be ingested into large language models or generative AI offerings without OpenStax's permission.
Want to cite, share, or modify this book? This book uses the Creative Commons Attribution License and you must attribute OpenStax.
Access for free at https://openstax.org/books/biology-2e/pages/1-introduction
- Authors: Mary Ann Clark, Matthew Douglas, Jung Choi
- Publisher/website: OpenStax
- Book title: Biology 2e
- Publication date: Mar 28, 2018
- Location: Houston, Texas
- Book URL: https://openstax.org/books/biology-2e/pages/1-introduction
- Section URL: https://openstax.org/books/biology-2e/pages/1-1-the-science-of-biology
© Apr 26, 2024 OpenStax. Textbook content produced by OpenStax is licensed under a Creative Commons Attribution License . The OpenStax name, OpenStax logo, OpenStax book covers, OpenStax CNX name, and OpenStax CNX logo are not subject to the Creative Commons license and may not be reproduced without the prior and express written consent of Rice University.
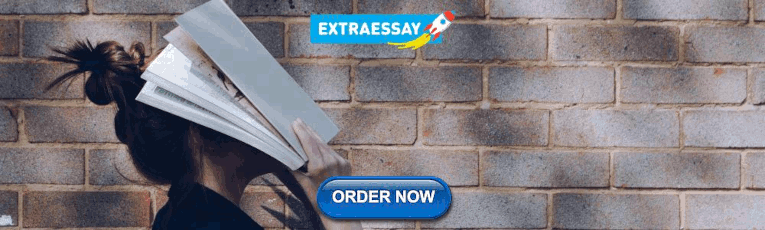
IMAGES
VIDEO
COMMENTS
The generation of submarine hydrothermal vents through meteorite impacts also circumvents a major problem for this origin-of-life hypothesis; namely that it is very uncertain as to whether black and white smoker-type environments—which at the present-day are associated with mid-ocean ridges—would have existed on early Earth due to the ...
The concentration of nucleobases in the Murchison meteorite is relatively low. Martins and her co-workers needed 0.5 ounce (15 grams) of space rock to extract their sample, compared with milligram ...
Panspermia proposes that organisms such as bacteria, complete with their DNA, could be transported by means such as comets through space to planets including Earth.. Panspermia (from Ancient Greek πᾶν (pan) 'all', and σπέρμα (sperma) 'seed') is the hypothesis that life exists throughout the Universe, distributed by space dust, meteoroids, asteroids, comets, and planetoids, as well as ...
The original meteorite is almost completely replaced by diagenetic minerals, primarily clay minerals, calcite, and barite. The only common relict mineral is chrome spinel. The Österplana 065 meteorite is the only fossil meteorite found in the Thorsberg quarry that is not an equilibrated ordinary chondrite.
Table 18.9.1 18.9. 1: Representative amino acids found in the Murchison meteorite. Six of the amino acids (blue) are found in all living things, but the others (yellow) are not normally found in living matter here on earth. The same amino acids are produced in discharge experiments like Miller's.
An enduring mystery regarding the origin of life is how biology could have arisen from non-biological chemical processes. DNA is the template for life, carrying the instructions for how to build and operate a living organism. However, RNA also carries information, and many researchers think it evolved first and was later replaced by DNA.
Introduction. Since Chladni (1794) published On the Origin of the Pallas Iron and Others Similar to it, and on Some Associated Natural Phenomena and made plausible the hypothesis that rocks could fall from the sky, the definition of the word meteorite has remained essentially unchanged, as reflected in the ten quotations given above. Nearly all modern reference works use a similar definition.
4 Department of Biology, Georgetown University, Washington, DC, USA. ... In the context of previously proposed environments for the origin of life on Earth, we discuss how meteorite impacts can generate both subaerial and submarine hydrothermal vents, abundant hydrothermal-sedimentary settings, and impact analogues for volcanic pumice rafts and ...
A comprehensive definition (from Rubin and Grossman 2014) is a meteorite is a natural, solid object larger than 10 μm in size, derived from a celestial body, that was transported by natural means from the body on which it formed to a region outside the dominant gravitational influence of that body and that later collided with a natural or ...
Meteorite: The Stones From ... Senior Postdoctoral Research Fellow required to lead exciting projects in Cancer Cell Cycle Biology and Cancer Epigenetics. Melbourne University, Melbourne (AU)
First published 26 April 2022. 52. Meteorites could have been responsible for delivering the basis of life's genetic code. Analyses of three meteorites suggest that nucleobases, the crucial components of DNA, could have formed in space and then fallen to Earth to provide the raw material for the origin of life itself.
He was the perfect person, because meteorites, undoubtedly you cannot talk meteorites without talking astronomy and the solar system. At the same time, the work is basically analytical chemistry. You know, I would say John Cronin, and then there's the Arizona State University meteorite center, I think the world's largest university meteorite ...
The evidence supporting this hypothesis is the finding in the Murchison meteorite of comparable suites of α-amino and α-hydroxy linear acids (although this correspondence is not valid for the α-methyl compounds) and of imino acids (e.g., Pizzarello and Cooper 2001).
In this article, we'll examine scientific ideas about the origin of life on Earth. The when of life's origins (3.5 billion years ago or more) is well-supported by fossils and radiometric dating. But the how is much less understood. In comparison to the central dogma or the theory of evolution, hypotheses about life's origins are much more...hypothetical.
An enduring mystery regarding the origin of life is how biology could have arisen from non-biological chemical processes, called prebiotic chemistry. ... Finding HMT in meteorites confirms the hypothesis that it is a stable source for ammonia and formaldehyde in asteroids." ... Daniel Glavin gets ready to powder the Murchison meteorite in a ...
The new discovery adds to the growing list of biologically important compounds that have been found in meteorites, supporting the hypothesis that chemical reactions in asteroids - the parent bodies of many meteorites - can make some of life's ingredients. If correct, meteorite bombardment on ancient Earth may have assisted the origin of ...
meteorite, any fairly small natural object from interplanetary space—i.e., a meteoroid —that survives its passage through Earth's atmosphere and lands on the surface. In modern usage the term is broadly applied to similar objects that land on the surface of other comparatively large bodies. For instance, meteorite fragments have been ...
Confirmation that a large meteorite impact in Mexico correlates with a mass extinction (about 76% of fossilizable species), including the dinosaurs, is revolutionizing geology. ... the Alvarez impact hypothesis generated a tremendous amount of exciting and productive research. ... and Department of Earth and Space Sciences, Molecular Biology ...
made huge diversity of animal species. What is the mesozoic era known as. the age of reptiles. When was the mesozoic era. began: 248 million years ago. ended: 65 million years ago. Some major events of the mesozoic era. dinosaurs, birds, first mammals,and flowering plants appeared. When was the cenozoic era.
Meteoroids are lumps of rock or metal that orbit the sun. Meteoroids become meteors when they crash into Earth's atmosphere and the gases surrounding them briefly light up as "shooting stars.". While most meteors burn up and disintegrate in the atmosphere, many of these space rocks reach Earth's surface in the form of meteorites.
Like geology, physics, and chemistry, biology is a science that gathers knowledge about the natural world. Specifically, biology is the study of life. The discoveries of biology are made by a community of researchers who work individually and together using agreed-on methods. In this sense, biology, like all sciences is a social enterprise like ...
A second hypothesis might be, "The classroom is warm because there is a power failure, and so the air conditioning doesn't work." Once one has selected a hypothesis, the student can make a prediction. A prediction is similar to a hypothesis but it typically has the format "If . . . then . . . ."
describe the hypothesis that says that RNA is the genetic material that stores information in living things on early Earth. thomas cech and sidney Altman discovered that RNA can catalyze their own replication and synthesis through ribozymes. Study with Quizlet and memorize flashcards containing terms like Nebula, describe the Miller-Urey ...