Recent Advances in the Treatment of Colorectal Cancer: A Review
Affiliation.
- 1 Departments of Gastrointestinal and Hepato-Biliary-Pancreatic Surgery, Nippon Medical School.
- PMID: 35082204
- DOI: 10.1272/jnms.JNMS.2022_89-310
Colorectal cancer (CRC) is the third most common cancer worldwide, and surgical treatment remains the first-line treatment to provide a cure. In addition to the aging population, obesity, low physical activity, and smoking habits increase CRC risk. Despite advances in surgical techniques, chemotherapy, and radiotherapy, colorectal cancer remains the second leading cause of cancer-related deaths worldwide. For early-stage CRC, endoscopic treatment, including endoscopic mucosal resection and endoscopic submucosal dissection, has been performed. However, lymph node dissection is an integral part of surgical treatment for advanced-stage cancer because of the high incidence of lymph node metastasis. Conventional open surgery has evolved into laparoscopic and robotic surgery. Although prospective studies have confirmed the safety and feasibility of laparoscopic surgery for CRC, relevant treatment models of transverse colon cancer and rectal cancer still need to be further explored and validated. Furthermore, multidisciplinary treatment is needed to cure CRC completely. This review aimed to provide an update on recent advances in the surgical treatment of CRC.
Keywords: chemotherapy; colorectal cancer; endoscopy; navigation; surgery.
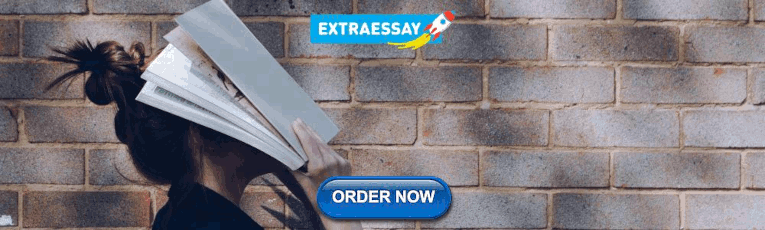
Publication types
- Colorectal Neoplasms* / surgery
- Laparoscopy*
- Lymph Node Excision / methods
- Lymphatic Metastasis
- Prospective Studies
- Rectal Neoplasms* / surgery
SYSTEMATIC REVIEW article
The histological and molecular characteristics of early-onset colorectal cancer: a systematic review and meta-analysis.
- 1 School of Medicine and Public Health, Carbone Cancer Center, University of Wisconsin-Madison, Madison, WI, United States
- 2 School of Medicine and Public Health, Department of Population Health Sciences, University of Wisconsin-Madison, Madison, WI, United States
Background: Early-onset colorectal cancer (CRC), defined as diagnosis before age 50, has increased in recent decades. Although more often diagnosed at advanced stage, associations with other histological and molecular markers that impact prognosis and treatment remain to be clarified. We conducted a systematic review and meta-analysis concerning the prevalence of prognostic and predictive tumor markers for early- vs. late-onset CRC, including oncogene mutations, microsatellite instability (MSI), and emerging markers including immune cells and the consensus molecular subtypes.
Methods: We systematically searched PubMed for original research articles published between April 2013–January 2024. Included studies compared the prevalence of tumor markers in early- vs. late-onset CRC. A meta-analysis was completed and summary odds ratios (ORs) with 95% confidence intervals (CIs) were obtained from a random effects model via inverse variance weighting. A sensitivity analysis was completed to restrict the meta-analysis to studies that excluded individuals with Lynch syndrome, a hereditary condition that influences the distribution of tumor markers for early-onset CRC.
Results: In total, 149 articles were identified. Tumors from early-onset CRC are less likely to include mutations in KRAS (OR, 95% CI: 0.91, 0.85-0.98), BRAF (0.63, 0.51-0.78), APC (0.70, 0.58-0.84), and NRAS (0.88, 0.78-1.00) but more likely to include mutations in PTEN (1.68, 1.04-2.73) and TP53 (1.34, 1.24-1.45). After limiting to studies that excluded Lynch syndrome, the associations between early-onset CRC and BRAF (0.77, 0.64-0.92) and APC mutation (0.81, 0.67-0.97) were attenuated, while an inverse association with PIK3CA mutation was also observed (0.88, 0.78-0.99). Early-onset tumors are less likely to develop along the CpG Island Methylator Phenotype pathway (0.24, 0.10-0.57), but more likely to possess adverse histological features including high tumor grade (1.20, 1.15-1.25), and mucinous (1.22, 1.16-1.27) or signet ring histology (2.32, 2.08-2.57). A positive association with MSI status (1.31, 1.11-1.56) was also identified. Associations with immune markers and the consensus molecular subtypes are inconsistent.
Discussion: A lower prevalence of mutations in KRAS and BRAF is consistent with extended survival and superior response to targeted therapies for metastatic disease. Conversely, early-onset CRC is associated with aggressive histological subtypes and TP53 and PTEN mutations, which may serve as therapeutic targets.
1 Introduction
Colorectal cancer (CRC) is the second leading cause of cancer mortality in the United States ( 1 ). The incidence of CRC has steadily declined since the 1980s, largely attributed to greater uptake of colonoscopy screening by adults aged 50 years and older ( 2 ). Concurrently, the incidence of sporadic early-onset CRC, generally defined as CRC diagnosis before age 50 without an underlying hereditary cause, has significantly increased since the mid-1990s ( 2 ). Data from the Surveillance, Epidemiology, and End Results (SEER) program reflect a 2-3% annual increase in the incidence of early-onset CRC ( 3 ). The elevated incidence of early-onset CRC may be explained by birth cohort effects where more recent birth cohorts have increased prevalence of obesity and type 2 diabetes, lower levels of physical activity, and more often consume western-style diets characterized by lower consumption of fruits and vegetables ( 4 ), as well as changes in the composition of the gut microbiome ( 2 ). While early-onset CRC may be caused by hereditary conditions defined by germline mutations in DNA mismatch-repair genes (i.e. Lynch syndrome) or in the tumor suppressor APC (i.e. familial adenomatous polyposis) ( 5 ), these inherited conditions account for a relatively small percentage of early-onset CRC and do not explain the increased prevalence observed in recent decades ( 2 ).
CRC is a heterogeneous disease and the clinicopathological and molecular characteristics of tumors may influence prognosis and response to treatment ( 6 ). Beyond tumor stage, multiple potential prognostic and predictive markers have been identified, including mutations in oncogenes such as KRAS , BRAF , PIK3CA , and TP53 , histological subtypes including mucinous and signet ring carcinomas, and the microsatellite instability (MSI) phenotype ( 7 ). Further, several novel prognostic markers have recently been identified, including immune markers in the tumor microenvironment ( 8 ) and the CRC consensus molecular subtypes ( 9 ). It is anticipated that the continued characterization of molecular phenotypes in CRC will augment traditional clinical markers for therapeutic decision making and support the development of targeted approaches to treatment ( 10 ).
Given the increasing rate of early-onset CRC, recent publications have highlighted potential differences in the clinicopathological and molecular characteristics of tumors based on age of onset ( 11 – 14 ). However, it is currently unclear whether early-onset CRC is distinct from late-onset disease in terms of molecular characteristics and tumor developmental pathways ( 15 ). Understanding the molecular characteristics of early-onset CRC is necessary to guide the development of therapeutic approaches for this condition and to address underlying causes. Therefore, we have completed a systematic review and meta-analysis to comprehensively summarize the evidence linking early-onset CRC to differences in prognostic and predictive tumor markers, including oncogene mutations, histological subtypes, MSI status, as well as anti-tumor immunity and the consensus molecular subtypes.
2.1 Literature review
Articles for this systematic review were identified utilizing a Pubmed search incorporating PRISMA guidelines ( 16 ). Given the wide breadth of the topic and the limited number of relevant articles published prior to 2013, the search was limited to peer-reviewed, original research articles published in English from the last 10 years (April 2013 – April 2023), with relevant keywords and medical subject headings included in the title and/or abstract. The literature review was repeated in January 2024 to identify recently published articles. Specific biomarker terms to include in the literature search were identified from prior reviews, and the search terms “biomark*”, “mark*”, and “character*” were included to capture potentially novel prognostic markers. All search terms included for the literature review are displayed in Supplementary Table S1 . Manuscripts were included that reported the prevalence of prognostic biomarkers in CRC tumors separately for early- vs. late-onset disease. Articles were excluded if the prevalence of tumor clinicopathological or molecular biomarkers were not provided for participants with CRC (see Figure 1 flowchart), or if there was no comparison between early- vs. late-onset CRC (or if the comparison was limited to tumor stage or location only). Articles were also excluded that described hereditary CRC only (e.g. Lynch syndrome), site-specific metastases, or included non-CRC cancers in the analysis samples. For the purposes of this analysis, early-onset disease was defined as CRC diagnosed prior to age 50. To avoid misclassification of early- and late-onset CRC, we excluded papers where late-onset CRC was defined as ≥ 40 years at diagnosis or younger, or where early-onset CRC was defined as ≤ 60 years at diagnosis or older. Lastly, to limit sample overlap where possible, we excluded studies if there was evidence of complete overlap in sample and markers reported with a previously published study, or if a study reported the same outcome in a subsample of a previous study.
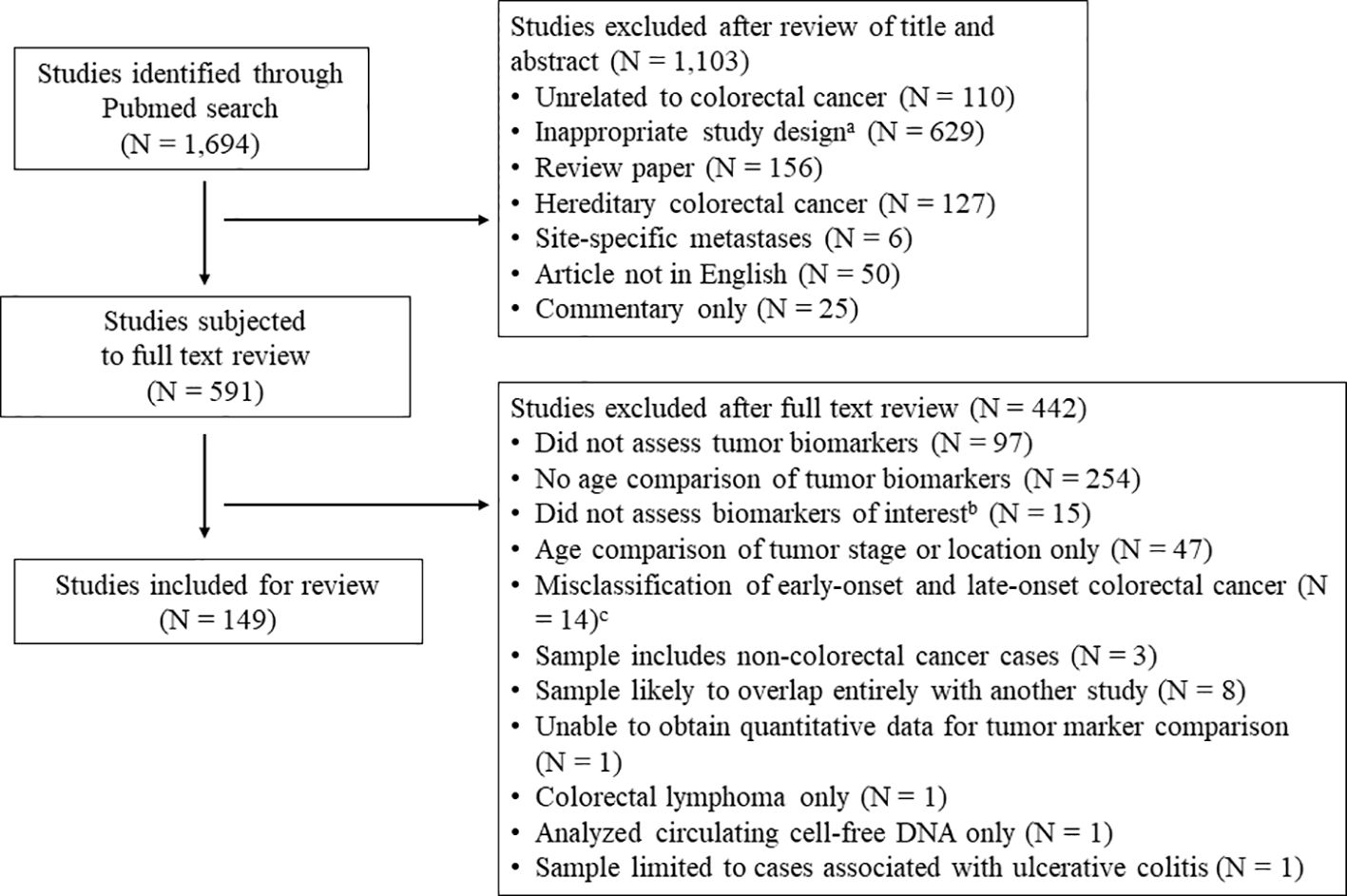
Figure 1 Literature review flowchart. a Inappropriate study design includes studies concerning colorectal cancer incidence, colonoscopy or other colorectal cancer screening, population level summary statistics for colorectal cancer, and studies of colorectal cancer in model organisms or in vitro studies. b Markers of interest include oncogene mutations in KRAS , NRAS , BRAF , PIK3CA , PTEN , TP53 , APC , and HER2 ; histological phenotypes including high-grade tumors and mucinous or signet ring histology; molecular carcinogenesis pathways including microsatellite instability and the CpG island methylator phenotype (CIMP); and novel tumor prognostic phenotypes including immune markers in the tumor microenvironment and the consensus molecular subtypes. c Studies where late-onset colorectal cancer was defined as ≥ 40 years at diagnosis (or younger), or early-onset CRC was defined as ≤ 60 years at diagnosis (or older).
The systematic review and meta-analysis was limited to the following markers that have been shown associations with CRC survival and/or therapeutic response in CRC: oncogene mutations in KRAS ( 17 – 20 ), NRAS ( 17 , 21 , 22 ), BRAF ( 17 , 19 , 23 , 24 ), PIK3CA ( 17 , 25 , 26 ), PTEN ( 27 , 28 ), TP53 ( 29 ), APC ( 30 , 31 ), and HER2 amplifications ( 32 – 34 ); histological phenotypes including high-grade tumors ( 35 , 36 ) and mucinous ( 37 , 38 ) or signet ring histology ( 38 , 39 ); molecular carcinogenesis pathways including MSI ( 40 ) and the CpG island methylator phenotype (CIMP) ( 41 ); and novel tumor prognostic phenotypes including immune markers ( 42 , 43 ) in the tumor microenvironment and the consensus molecular subtypes ( 9 , 44 ). Because it is well-established that early-onset CRC is associated with advanced tumor summary stage at diagnosis and rectal tumor location, these markers are not summarized in this review. The literature review was completed by two authors (T.L. and L.P) independently. Disagreements between reviewers were resolved by further review of the manuscript to determine whether the study included a comparison of tumor markers of interest between early- and late-onset CRC. The final decision to include a manuscript was made by the lead author. In total, 1,694 articles were identified from the literature search and 149 were eligible for review ( Figure 1 ). For each study, the potential for bias was evaluated by the lead author using the Newcastle-Ottawa Scale adapted for cross-sectional studies ( 45 ). Pre-registration of the systematic review protocol was not performed.
2.2 Meta-analysis
From each eligible study, the number of mutant and wild-type tumors for each marker in early- and late-onset CRC was extracted by the lead author. Data extraction was completed in duplicate, and the results from the two extractions were compared to identify any errors or inconsistencies in the sample sizes, which were subsequently revised after further review of the original article. If these data were not available from the manuscript, sample sizes were requested from the corresponding author. One study was excluded for which we were unable to obtain the necessary sample sizes from each group ( 46 ). When necessary, sample sizes for separate age groups were combined to create a single category for early-onset and late-onset CRC. For most studies, age 45 or 50 at diagnosis was utilized as the threshold to distinguish early- vs. late-onset CRC, although occasionally other classifications were employed (see Supplementary S2 ). For each study, sample characteristics including overall sample size, country, tumor stage, sex, or other distinguishing features were also extracted. For each marker, an odds ratio (OR) and 95% confidence interval (CI) were calculated using a standard equation ( 47 ). For mutations in oncogenes KRAS, NRAS, BRAF, PIK3CA, PTEN, TP53 , and APC , as well as MSI status and histological subtypes, meta-analyses were completed to compare the prevalence in tumors from early- vs. late-onset CRC. Due to the wide variety of immune markers that have been reported, a meta-analysis was not attempted for the comparison of immune phenotypes in the tumor microenvironment. For each marker that was meta-analyzed, a pooled OR with 95% CI was obtained from a random effects model via inverse variance weighting. The random effects model was selected a priori , as between-study heterogeneity is plausible given variability in the definition of early-onset CRC, as well as differences in tumor location, race, nationality and stage between studies. The random effects meta-analysis is capable of providing unbiased estimates in the presence of heterogeneity and will generally provide more conservative estimates than the fixed-effects model (which assumes no between-study heterogeneity) ( 48 ). Heterogeneity was determined via the Cochrane’s Q statistic and the I 2 statistic. Significant heterogeneity was defined as P <.05 for Cochrane’s Q or I 2 ≥ 50%. To determine whether the meta-analysis estimates were influenced by a single study, a ‘leave-one-out’ sensitivity analysis was conducted for each marker. Because Lynch syndrome may influence the prevalence of tumor markers for individuals with early-onset CRC, a second sensitivity analysis was completed to limit the analysis to studies that specifically excluded individuals with Lynch syndrome or family history of CRC, or that restricted the sample to microsatellite stable tumors. All statistical tests were two-sided, with statistical significance defined using a threshold of P <.05. All meta-analyses were completed using Review Manager 5.4.1 from Cochrane.
In total, 149 articles were reviewed that compared the prevalence of clinicopathological tumor markers in early- vs. late-onset CRC. All meta-analysis results are summarized in Table 1 . Sample characteristics and references for all included studies are presented in Supplementary Table S2 . Results of the bias assessment utilizing the Newcastle-Ottawa Scale are presented in Supplementary Table S4 .
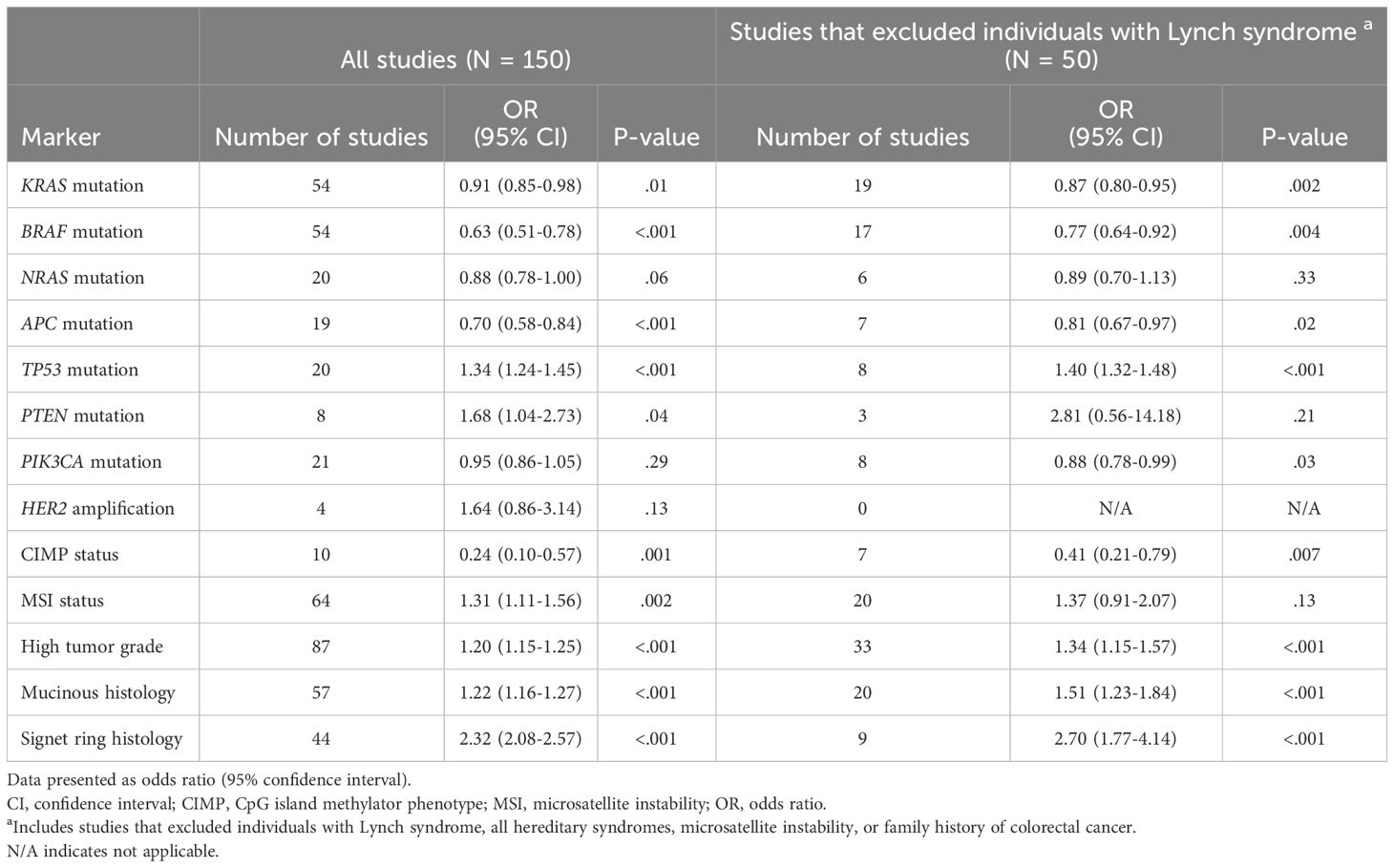
Table 1 Summary of meta-analysis results showing associations between early-onset colorectal cancer and the prevalence of tumor markers, compared to late-onset colorectal cancer.
3.1 Oncogene mutations
The number of studies identified for the following markers is as follows: KRAS mutation ( 49 ); BRAF mutation ( 49 ); NRAS mutation ( 20 ); PIK3CA mutation ( 21 ); PTEN mutation ( 8 ); HER2 amplifications ( 5 ); APC mutation ( 19 ); TP53 mutation ( 20 ). For early-onset CRC, there is evidence for a significantly lower prevalence of mutations in KRAS ( Figure 2 , OR 0.91, 95% CI 0.85-0.98), BRAF ( Figure 3 , OR 0.63, 95% CI 0.51-0.78) and APC ( Figure 4 , OR 0.70, 95% CI 0.58-0.84) compared to late-onset CRC. Early-onset CRC was associated with non-significantly lower prevalence of mutations in NRAS ( Figure 5 , OR 0.88, 95% CI 0.78-1.00, p = .06). Conversely, early-onset CRC is associated with a higher prevalence of mutations in TP53 ( Figure 6 , OR 1.34, 95% CI 1.24-1.45) and PTEN ( Figure 7 , OR 1.68, 95% CI 1.04-2.73). There was no significant difference in the prevalence of PIK3CA mutations ( Supplementary Figure S1 , OR 0.95, 95% CI 0.86-1.05), or HER2 amplifications ( Supplementary Figure S2 , OR 1.64, 95% CI 0.86-3.14). Significant inter-study heterogeneity was observed for mutations in KRAS , BRAF , PTEN , and APC . Hazard ratios for oncogene mutations were stable in the leave-one-out sensitivity analysis ( Supplementary Table S3 ), although the association for NRAS and PTEN mutations did not always reach statistical significance.
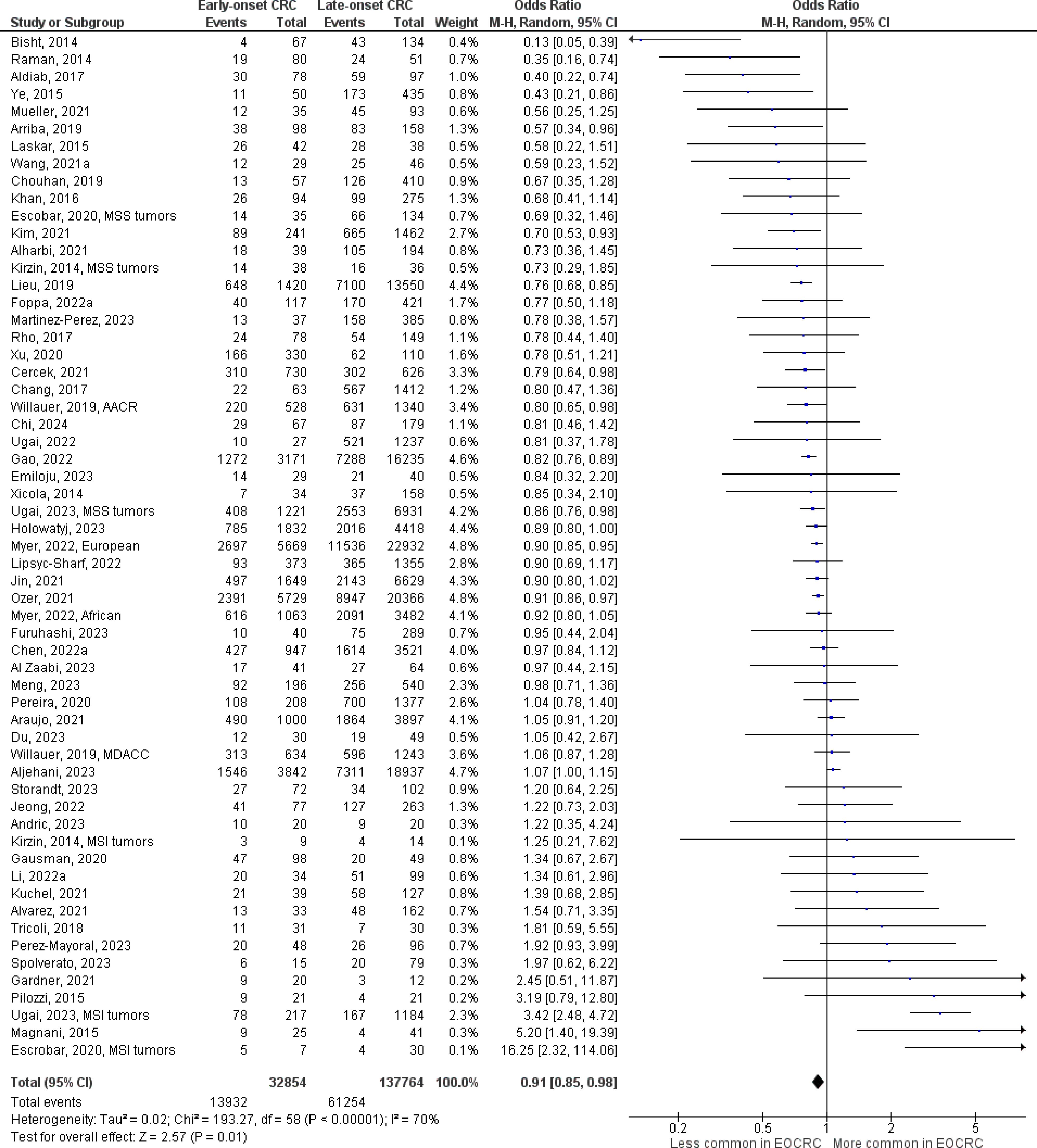
Figure 2 Odds ratios for KRAS mutation in early-onset CRC. Data presented as odds ratios (95% confidence interval) for KRAS mutation in early-onset relative to late-onset colorectal cancer. The pooled odds ratio is obtained via a random effects model using inverse variance weighting. AACR, American Association for Cancer Research; MDACC, MD Anderson Cancer Center; MSI, microsatellite instability; MSS, microsatellite stable.
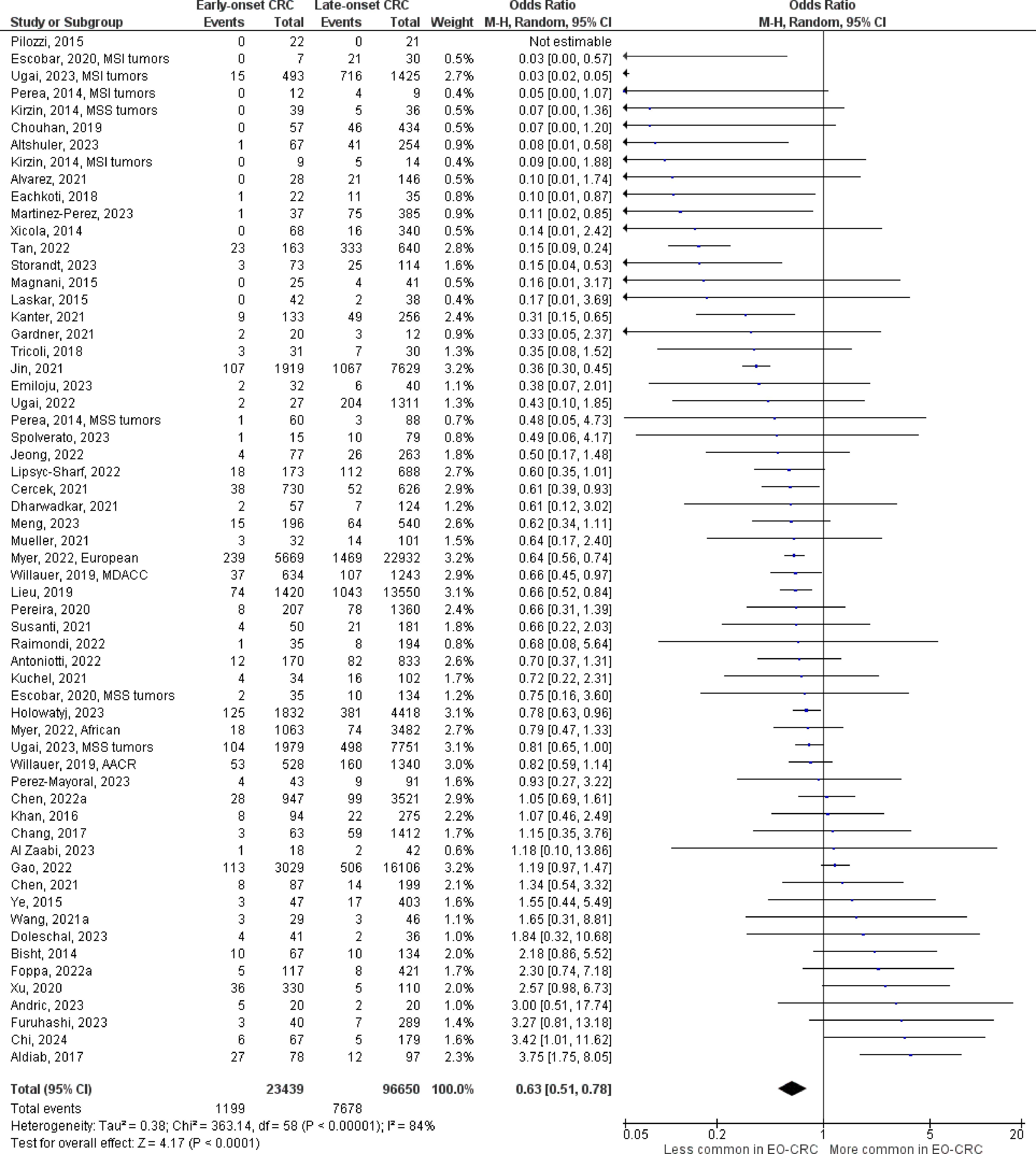
Figure 3 Odds ratios for BRAF mutation in early-onset CRC. Data presented as odds ratios (95% confidence interval) for BRAF mutation in early-onset relative to late-onset colorectal cancer. The pooled odds ratio is obtained via a random effects model using inverse variance weighting. AACR, American Association for Cancer Research; MDACC, MD Anderson Cancer Center; MSI, microsatellite instability; MSS, microsatellite stable.
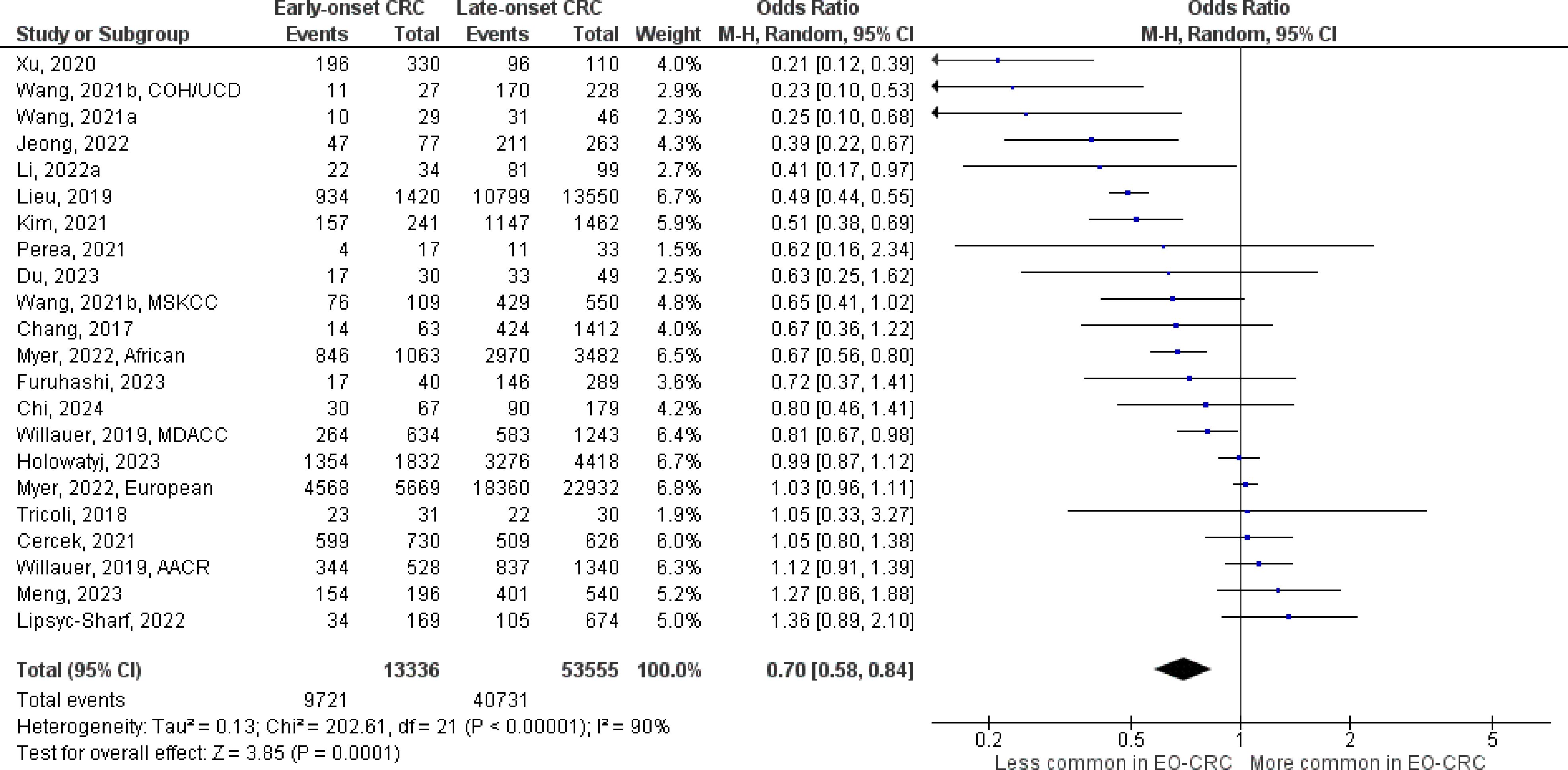
Figure 4 Odds ratios for APC mutation in early-onset colorectal cancer. Data presented as odds ratios (95% confidence interval) for APC mutation in early-onset relative to late-onset colorectal cancer. The pooled odds ratio is obtained via a random effects model using inverse variance weighting. AACR, American Association for Cancer Research; COH, City of Hope National Medical Center; CI, confidence interval; EO-CRC, early-onset colorectal cancer; MDACC, MD Anderson Cancer Center; MSKCC, Memorial Sloan Kettering Cancer Center; UCD, University of California, Davis.
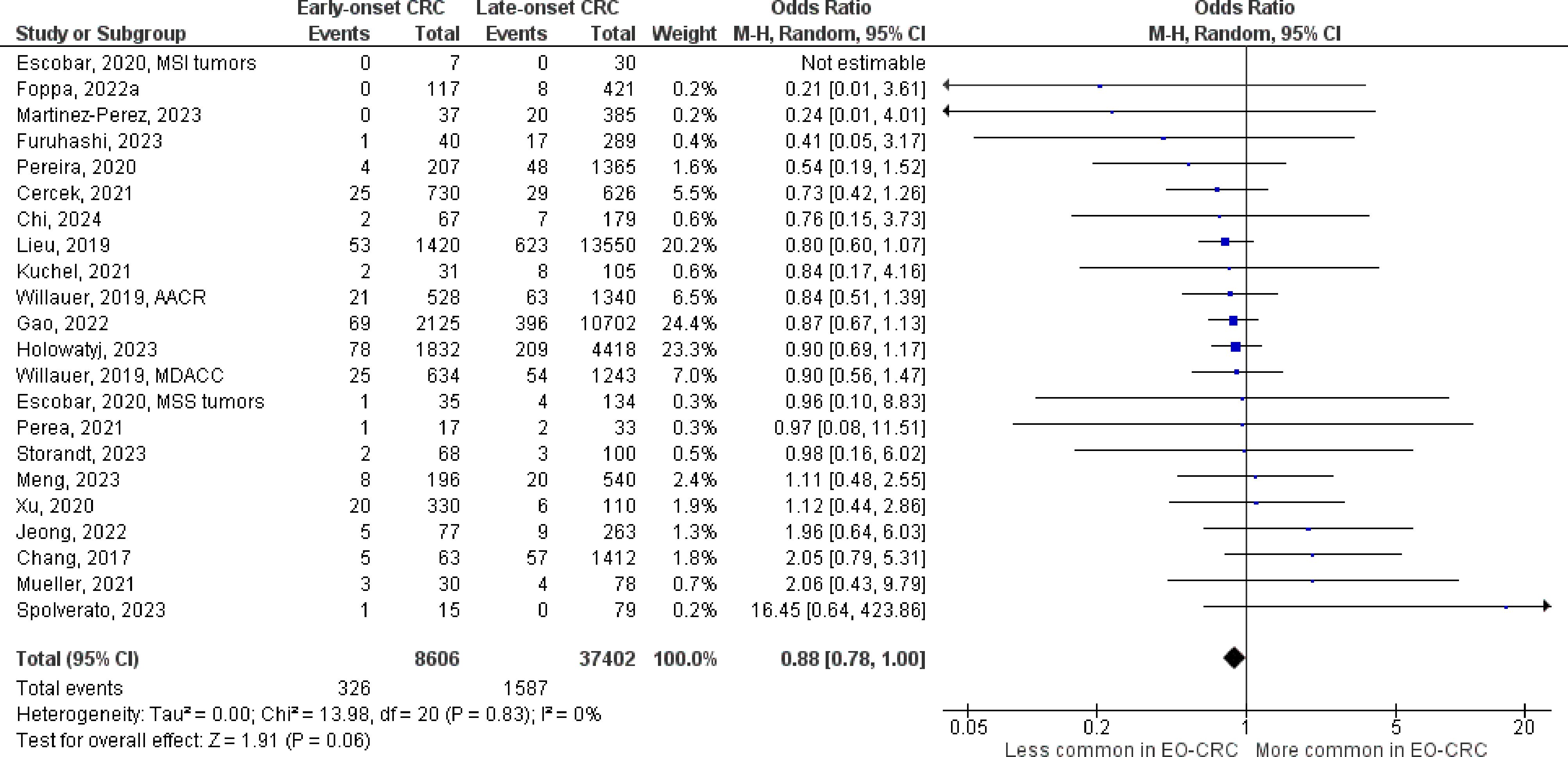
Figure 5 Odds ratios for NRAS mutation in early-onset colorectal cancer. Data presented as odds ratios (95% confidence interval) for NRAS mutation in early-onset relative to late-onset colorectal cancer. The pooled odds ratio is obtained via a random effects model using inverse variance weighting. AACR, American Association for Cancer Research; CI, confidence interval; EO-CRC, early-onset colorectal cancer; MDACC, MD Anderson Cancer Center.
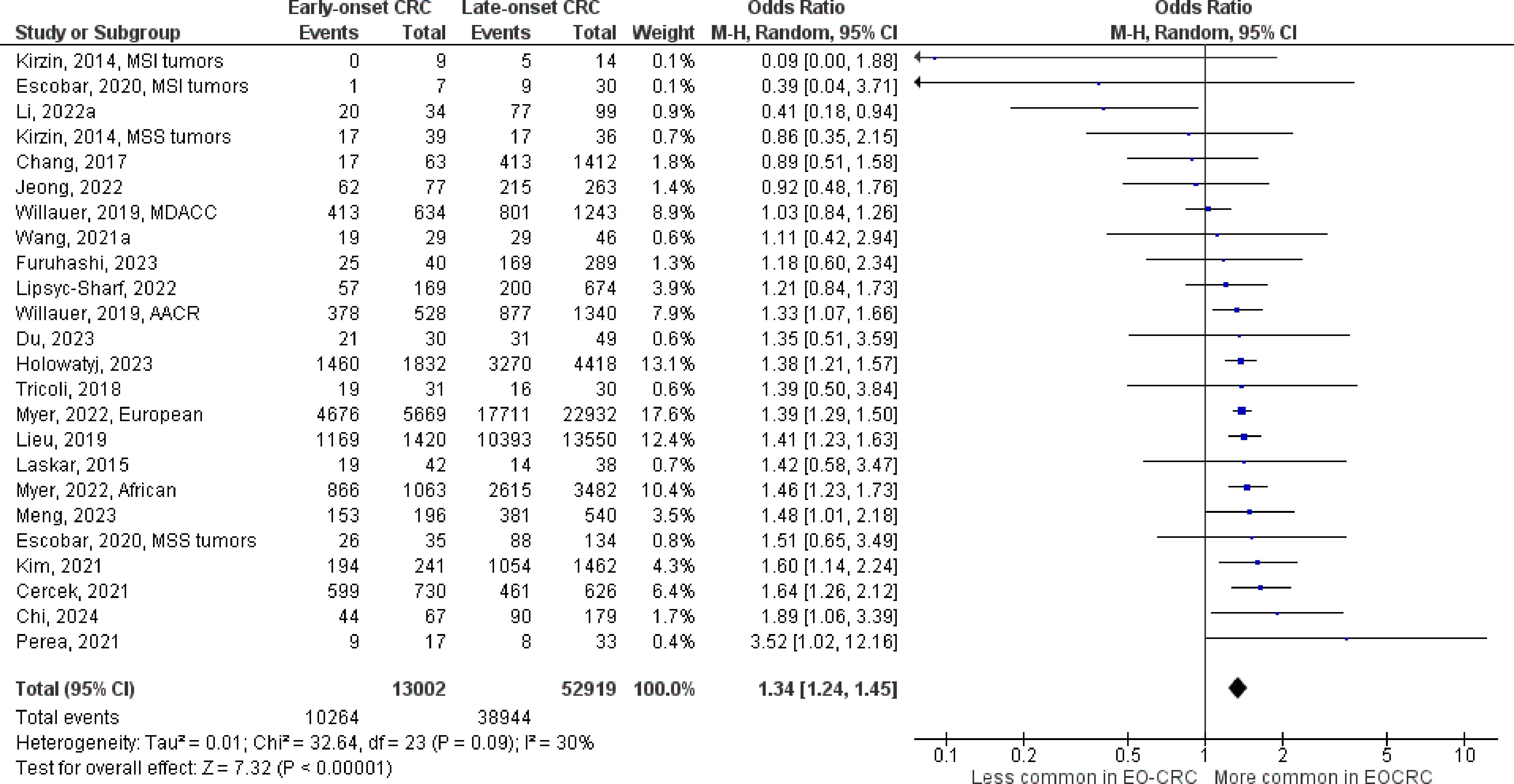
Figure 6 Odds ratios for TP53 mutation in early-onset colorectal cancer. Data presented as odds ratios (95% confidence interval) for TP53 mutation in early-onset relative to late-onset colorectal cancer. The pooled odds ratio is obtained via a random effects model using inverse variance weighting. AACR, American Association for Cancer Research; CI, confidence interval; EO-CRC, early-onset colorectal cancer; MDACC, MD Anderson Cancer Center; MSI, microsatellite instability; MSS, microsatellite stability.
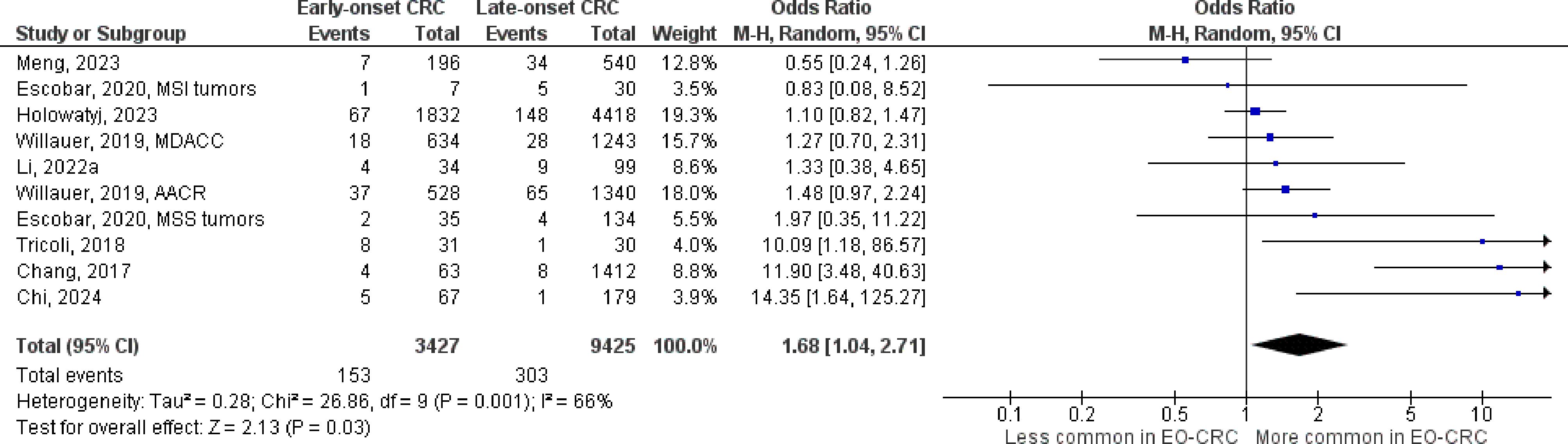
Figure 7 Odds ratios for PTEN mutation in early-onset colorectal cancer. Data presented as odds ratios (95% confidence interval) for PTEN mutation in early-onset relative to late-onset colorectal cancer. The pooled odds ratio is obtained via a random effects model using inverse variance weighting. AACR, American Association for Cancer Research; CI, confidence interval; EO-CRC, early-onset colorectal cancer; MDACC, MD Anderson Cancer Center.
Fifty studies were identified that specifically excluded individuals with Lynch syndrome or family history of CRC, or that restricted the analysis to individuals with microsatellite stable tumors ( Table 1 ; Supplementary Table S2 ). Compared to the full analysis, the association between early-onset CRC and BRAF (OR 0.77, 95% CI 0.64-0.92) and APC mutations (OR 0.81, 95% CI 0.67-0.97) were attenuated but remained statistically significant, while the associations with KRAS , NRAS , and TP53 mutations were similar. Further, an inverse association between early-onset CRC and PIK3CA mutation was also observed (OR 0.88, 95% CI 0.78-0.99).
3.2 Molecular carcinogenesis pathways
There were 10 studies that compared the prevalence of CIMP-high status in early- vs. late-onset CRC, and 64 studies that compared MSI status. Individuals with early-onset CRC had significantly lower odds for CIMP-high tumors compared to individuals with late-onset disease ( Supplementary Figure S3 , OR 0.24, 0.10-0.57), but significantly higher odds for the MSI phenotype ( Supplementary Figure S4 , OR 1.31, 1.11-1.56). Significant heterogeneity was observed for both markers. Associations were stable in the leave-one-out sensitivity analysis ( Supplementary Table S3 ), and after limiting the analysis to studies that excluded individuals with Lynch syndrome or family history of CRC ( Table 1 ).
3.3 Histological characteristics
There were 86 studies that compared the prevalence of high-grade tumors (i.e. poorly differentiated or undifferentiated tumors) in early- vs. late-onset CRC, 57 studies that compared the prevalence of mucinous histology (or mucinous characteristics), and 44 studies that reported on signet ring cell carcinomas. In early-onset CRC, there was evidence for a significantly higher prevalence of high-grade (i.e., poorly differentiated) tumors ( Supplementary Figure S5 , OR 1.20, 95% CI 1.15-1.25), as well as mucinous tumors ( Supplementary Figure S6 , OR 1.22, 95% CI 1.16-1.27), and signet ring cell carcinomas ( Supplementary Figure S7 , OR 2.32, 2.08-2.57). Significant inter-study heterogeneity was observed for all histological markers. All associations were stable in the leave-one-out sensitivity analysis ( Supplementary Table S3 ) and after limiting the analysis to studies that excluded individuals with Lynch syndrome or family history of CRC ( Table 1 ).
3.4 Immune markers
There have been nine studies to investigate age differences in the immune cell populations of CRC tumors, with inconsistent results ( 49 – 57 ). Du et al. reported that Chinese patients with sporadic early-onset CRC showed significantly higher densities of multiple immune cell populations in the tumor microenvironment compared to patients with late-onset disease, including higher levels of B cells, CD4+ T cells, CD8+ T cells, neutrophils, macrophages, and dendritic cells ( 50 ). By contrast, Ugai et al. reported no significant differences in the populations of T cells, macrophages, and other myeloid cells in participants with early- vs. late-onset CRC from the Nurses’ Health Study and Health Professionals Follow-up Study ( 51 ). In a small study of 14 tumors utilizing single cell RNA sequencing, Li et al. reported that early-onset CRC was associated with lower levels of effector CD8+ T cells and antigen-presentation in the tumor microenvironment, but higher levels of naïve CD8+ T cells and immunosuppressive regulatory T cells compared to individuals with late-onset disease, suggesting an impaired anti-tumor immune response for early-onset CRC ( 54 ). Because MSI status may influence the anti-tumor immune response, recent studies have examined associations between early-onset CRC and tumor lymphocyte populations in samples limited to microsatellite stable tumors, or after careful exclusion of participants with Lynch syndrome ( 56 , 57 ). In a matched analysis of microsatellite stable tumors, Lu et al. (2023) reported that there was no significant differences between early- and late-onset CRC for the infiltration of 22 different lymphocyte populations in the tumor microenvironment ( 57 ). Likewise, Andric et al. found no significant difference for five lymphocyte populations (total T cells, conventional CD4+ and CD8+ T cells, regulatory T cells, and γδ T cells) in a matched sample limited to cases of sporadic CRC ( 56 ). Other studies have reported no significant differences between early and late-onset CRC for the density of total tumor infiltrating lymphocytes ( 53 , 55 ).
3.5 The consensus molecular subtypes
There have been six studies to determine the distribution of consensus molecular subtypes (CMS) for CRC by age at diagnosis ( 50 , 57 – 61 ). Utilizing tumor tissues samples from 626 individuals diagnosed with CRC from The Cancer Genome Atlas and MD Anderson Cancer Center, Willauer reported that the CMS1 subtype was more common among patients aged 30-39 years at diagnosis (46%) compared to older participants, while the CMS4 subtype was less common (13%) ( 58 ). Conversely, in a smaller study from the Nanjing Colorectal Cancer Cohort, Du et al. reported a higher prevalence of the CMS4 subtype in early- vs. late-onset CRC (36.7% vs. 12.2%, respectively), although the comparison between age groups did not reach statistical significance ( 50 ). Recent results, including from a small sample of South Korean participants ( 59 ) and additional analyses of The Cancer Genome Atlas ( 60 , 61 ) did not show any significant association between early-onset tumors and the distribution of consensus molecular subtypes.
4 Discussion
Sporadic early-onset CRC is a significant public health concern, increasing by 2-3% per year in the U.S. since 1990 ( 3 , 62 ). Early-onset CRC is more often diagnosed at advanced stages compared to late-onset disease ( 63 , 64 ). However, there is inconsistent evidence that survival varies between early- and late-onset CRC ( 65 , 66 ), complicated by reports that younger patients receive more aggressive systemic treatment ( 67 – 69 ). Thus, international guidelines do not endorse separate treatment recommendations for early-onset disease ( 70 ). Investigating the associations between early-onset tumors and molecular and histological characteristics, and novel tumor markers including immune cell populations, may help to guide the development of therapies that benefit early-onset CRC. Further, highlighting associations between early-onset CRC and tumor markers may aid in the design of clinical trials for targeted therapies. To the authors’ knowledge, this is the first comprehensive systematic review and meta-analysis of tumor prognostic and predictive markers in early-onset CRC. We found that early-onset CRC was associated with a lower prevalence of oncogene mutations in KRAS , BRAF , NRAS , and APC , but a higher prevalence of TP53 and PTEN mutations and adverse histologic subtypes, with inconsistent associations for immune cell populations and the consensus molecular subtypes.
KRAS , BRAF , and NRAS encode proteins that act downstream of the epidermal growth factor receptor (EGFR) and activate Mek/Erk signaling ( 21 , 71 ). Mutations in these oncogenes are negative predictive markers for EGFR inhibition in metastatic CRC ( 17 , 18 ) and are associated with inferior survival outcomes across tumor stage ( 19 , 20 , 23 , 72 ), including for early-onset CRC ( 73 – 75 ). Early-onset CRC is associated with a lower prevalence of mutations in these genes compared to late-onset disease, indicating that individuals with metastatic early-onset CRC may be more likely to benefit from EGFR inhibition. Notably, the association with NRAS mutations was not statistically significant, which may be due to the scarcity of this marker ( 76 ). Further, the association with BRAF mutation was attenuated but still statistically significant in studies that excluded individuals with Lynch syndrome, who are less likely to have BRAF mutations compared to sporadic disease ( 77 ). Further, this sensitivity analysis revealed an inverse association with PIK3CA mutation, which has also been linked to higher risk for mortality and resistance to EGFR inhibition ( 17 , 78 ). Conversely, early-onset CRC was associated with a higher proportion of mutations in tumor suppressor PTEN , which encodes a lipid-phosphatase that suppresses the activity of PI3k/Akt/mTOR signaling and interacts with the EGFR pathway ( 27 ). Loss of PTEN activity has been linked to resistance to EGFR inhibition in metastatic CRC ( 79 ) but is not currently used in clinical decision making. Pharmaceutical therapies to restore normal PTEN activity are under development but have not been evaluated in CRC. Early-onset CRC was associated with a significantly higher prevalence of TP53 mutations, which cause loss of p53 tumor suppressor activity and pro-tumorigenic gain of function effects that accelerate cell proliferation, angiogenesis, and metastasis ( 80 ). TP53 mutations are found in approximately 60% of tumors and may promote resistance to EGFR inhibitors and chemotherapies that rely on wild type p53 to induce cellular apoptosis (e.g. 5-fluorouracil and Oxaliplatin) ( 29 ). Consequently, targeted therapies to restore wild type p53 activity or degrade mutant p53, or to inhibit downstream effector pathways, are currently being investigated in clinical trials ( 81 ). Potentially, individuals with early-onset CRC may be more likely to benefit from treatments that inhibit pro-tumorigenic p53 activity and should be targeted for enrollment in these trials.
Early-onset CRC was associated with a lower prevalence of APC mutation, a key driver of the canonical adenoma-carcinoma pathway ( 82 ). APC mutations are present in approximately 80% of CRC tumors ( 11 , 12 , 14 ), and recent evidence indicates that APC -mutant tumors are associated with extended overall and progression-free survival compared to wild type ( 30 , 31 ) ( 5 ). Notably, the association with APC mutation was attenuated but still statistically significant when limiting the analysis to studies that excluded individuals with Lynch syndrome, or that included microsatellite stable tumors only. Individuals with early-onset CRC had a higher prevalence of MSI, defined by a high density of somatic mutations in short, non-coding sequences caused by defects in DNA mismatch repair ( 40 ). MSI is associated with lower risk for overall mortality and distant metastases compared to microsatellite stable tumors, including in early-onset CRC ( 75 ). Further, MSI tumors secrete truncated proteins that trigger an anti-tumor immune response ( 83 ), and consequently MSI is a positive predictor for response to immune checkpoint inhibitors ( 83 ). Our findings therefore highlight the importance of MSI testing for individuals younger than 50, in accordance with clinical guidelines ( 70 ). Unexpectedly, the association between early-onset CRC and MSI status was modestly strengthened in studies that excluded individuals with known Lynch syndrome, which causes tumors with MSI ( 84 ). Because a significant proportion of individuals with Lynch syndrome may be unaware of the condition ( 85 ), it is possible that the exclusion of Lynch syndrome was incomplete in some studies. Early-onset CRC was associated with a lower prevalence of the CpG island methylator phenotype (CIMP), characterized by methylation and inactivation of tumor-suppressor genes ( 86 ). Although CIMP has been linked to poor prognosis in multiple studies, it currently has limited value as a prognostic marker due to a lack of standardized assessment and competing effects of MSI and BRAF mutation, which are associated with CIMP ( 41 ).
We also found that early-onset CRC is associated with higher odds for tumors with more aggressive histological features, including poorly differentiated tumors, mucinous carcinomas, and signet ring cell carcinomas ( 38 , 87 ). The association with signet ring features was especially pronounced (OR [95% CI]: 2.32 [2.08-2.57]). Although signet ring carcinomas comprise only 1% of CRC tumors ( 39 ), this feature is present in 2-3% of early-onset tumors. A recent meta-analysis showed that signet ring carcinomas were associated with significantly higher risk for overall mortality and recurrence compared to conventional adenocarcinomas ( 88 ). Results were similar for mucinous tumors, which comprise approximately 10-15% of CRCs ( 89 ). The associations between histological subtypes and colorectal cancer mortality, especially poorly differentiated tumors and signet ring carcinomas, have been validated in early-onset CRC ( 90 – 93 ). Currently, there are no treatments that specifically target mucinous or signet ring cell carcinomas and treatment guidelines do not distinguish between histological subtypes ( 70 ).
The observed associations between early-onset CRC and certain histological and molecular tumor characteristics may be explained in part by differences in tumor location ( 94 ). Approximately 30% of early-onset tumors are located in the rectum, versus 20% of late-onset tumors ( 64 , 95 ). KRAS, BRAF, PIK3CA , and NRAS mutations are enriched in proximal tumors ( 96 , 97 ) while TP53 mutations are enriched in rectal tumors ( 98 ). Notably, studies that were limited to individuals with tumors in the distal colon or rectum have not shown a consistent association between early-onset CRC and the presence of oncogene mutations ( 46 , 55 , 56 , 99 – 102 ). For example, a study with more than 1,000 distal and rectal tumors showed no significant age difference in KRA S, BRAF, NRAS , PIK3CA , TP53 , or APC mutations ( 46 ). Conversely, in a large-scale analysis with detailed stratification by tumor location, Ugai et al. found that early-onset CRC had a lower prevalence of BRAF mutations for all tumor sites except the sigmoid colon and rectum ( 103 ). Notably, aggressive histological subtypes are overrepresented in the proximal colon ( 104 ), and consequently the association with early-onset CRC is not explained by differences in tumor location.
We found inconsistent evidence linking early-onset CRC to differences in ‘novel’ tumor prognostic and predictive markers including populations of immune cells in the tumor microenvironment ( 8 ). A recent meta-analysis demonstrated that a higher density of tumor infiltrating lymphocytes was associated with reduced overall mortality among 20,015 individuals with CRC (HR [95% CI]: 0.65 [0.54-0.77]) ( 42 ), while others have shown that an ‘immunoscore’ encompassing cytotoxic T cells and CD3+ cells was a superior prognostic marker compared to the tumor stage ( 105 , 106 ). Currently, the association between early-onset CRC and the anti-tumor immune response has been inconsistent ( 48 – 50 , 52 , 53 , 55 , 56 , 58 ). Notably, higher rates of MSI in early-onset CRC due to Lynch syndrome may obscure associations with immune markers in sporadic disease, as MSI tumors trigger a robust anti-tumor immune response ( 83 ). Studies limited to microsatellite stable tumors or that carefully excluded participants with hereditary syndromes have tended to show no significant differences in immune cell populations between early- and late-onset CRC ( 51 , 56 , 57 ). Likewise, there is currently no consistent evidence that the distribution of consensus molecular subtypes differs between early- and late-onset CRC, with most studies reporting null findings ( 50 , 57 , 59 – 61 ). The consensus molecular subtypes have shown to be a robust predictor of mortality outcomes independent of tumor stage ( 107 ), but to the authors’ knowledge have not been validated specifically in early-onset CRC. Further, the identification of novel molecular subtypes in early-onset CRC based on tumor gene expression is an area for future research.
Strengths of this study include the comprehensive nature of the search strategy, as we were able to summarize the evidence for age-related differences in the prevalence of established tumor prognostic markers as well as emerging markers including immune cell populations in the tumor microenvironment and the consensus molecular subtypes. Further, the large number of studies identified for most markers allowed for relatively precise estimates of the association with early-onset CRC. Lastly, to better understand the associations between early-onset CRC and tumor markers in sporadic disease, we completed a sensitivity analysis limited to studies that excluded individuals with known Lynch syndrome (or family history of CRC). This analysis is also attended by several limitations. Due to the breadth of the review, our literature search was limited to original research studies published within the last ten years in Pubmed. Consequently, it is possible that a relevant study was missed. However, this is unlikely to be a significant limitation given the paucity of large tumor genomic studies published prior to 2013 and the comprehensive nature of our search strategy. Further, there was evidence for significant heterogeneity in the estimates for most tumor markers, but we were unable to investigate underlying sources of inter-study heterogeneity because the prevalence of tumor prognostic markers was rarely presented in subgroups defined by tumor location, tumor stage, or MSI status. Between-study differences in the definitions of early- and late-onset CRC may also have contributed to heterogeneity, although we excluded studies where misclassification of early-onset CRC was apparent. Lastly, although we attempted to control for bias by performing a sensitivity analysis limited to studies that accounted for Lynch syndrome in the study design, it is possible that residual confounding by hereditary conditions or differences in tumor location may have biased the results.
5 Conclusions
In summary, early-onset CRC was associated with a lower prevalence of mutations in several oncogenes linked to mortality and poor therapeutic response, including KRAS , BRAF , and NRAS compared to individuals with late-onset disease. Conversely, early-onset disease was associated with a higher prevalence of potentially harmful mutations in TP53 and PTEN , as well as aggressive histological subtypes including mucinous and signet ring cell carcinomas. In part, these associations may reflect the higher prevalence of rectal tumors in early-onset CRC and the effect of hereditary syndromes on tumor markers. Given these findings and the alarming rise in the incidence of early-onset CRC, it is essential that clinical trials for targeted therapies enroll sufficient numbers of individuals with early-onset disease to evaluate their efficacy in this subgroup. Additional research is required to clarify the relationships with novel tumor characteristics including immune markers and to identify molecular subtypes specific to early-onset CRC that can inform treatment and prognosis.
Data availability statement
The original contributions presented in the study are included in the article/ Supplementary Material . Further inquiries can be directed to the corresponding author.
Author contributions
TL: Writing – review & editing, Writing – original draft, Visualization, Methodology, Investigation, Formal analysis, Data curation. LP: Writing – review & editing, Writing – original draft, Investigation, Data curation. SW: Writing – review & editing, Supervision, Resources, Project administration, Methodology, Funding acquisition, Conceptualization.
The author(s) declare financial support was received for the research, authorship, and/or publication of this article. This work was supported by the National Cancer Institute of the National Institutes of Health [NIH/NCI] under grants R00 CA207848 and R01 CA255318.
Conflict of interest
The authors declare that the research was conducted in the absence of any commercial or financial relationships that could be construed as a potential conflict of interest.
Publisher’s note
All claims expressed in this article are solely those of the authors and do not necessarily represent those of their affiliated organizations, or those of the publisher, the editors and the reviewers. Any product that may be evaluated in this article, or claim that may be made by its manufacturer, is not guaranteed or endorsed by the publisher.
Supplementary material
The Supplementary Material for this article can be found online at: https://www.frontiersin.org/articles/10.3389/fonc.2024.1349572/full#supplementary-material
Abbreviations
AACR, American Association for Cancer Research; APC, adenomatous polyposis coli; CI, confidence interval; CIMP, CpG island methylator phenotype; CMS, consensus molecular subtypes; COH, City of Hope (National Medical Center); CRC, colorectal cancer; EGFR, epidermal growth factor receptor; MDACC, MD Anderson Cancer Center; MSI, microsatellite instability; MSKCC, Memorial Sloan Kettering Cancer Center; MSS, microsatellite stable; OR, odds ratio; SEER, Surveillance, Epidemiology, and End Results; TIL, tumor infiltrating lymphocytes.
1. Siegel RL, Wagle NS, Cercek A, Smith RA, Jemal A. Colorectal cancer statistics, 2023. CA Cancer J Clin . (2023). 73(3):233–54. doi: 10.3322/caac.21772
PubMed Abstract | CrossRef Full Text | Google Scholar
2. Weinberg BA, Marshall JL. Colon cancer in young adults: trends and their implications. Curr Oncol Rep . (2019) 21:3. doi: 10.1007/s11912-019-0756-8
3. Murphy CC, Singal AG, Baron JA, Sandler RS. Decrease in incidence of young-onset colorectal cancer before recent increase. Gastroenterology . (2018) 155:1716–9. doi: 10.1053/j.gastro.2018.07.045
4. Done JZ, Fang SH. Young-onset colorectal cancer: A review. World J Gastrointest Oncol . (2021) 13:856–66. doi: 10.4251/wjgo.v13.i8.856
5. Ballester V, Rashtak S, Boardman L. Clinical and molecular features of young-onset colorectal cancer. World J Gastroenterol . (2016) 22:1736–44. doi: 10.3748/wjg.v22.i5.1736
6. Sagaert X, Vanstapel A, Verbeek S. Tumor heterogeneity in colorectal cancer: what do we know so far? Pathobiology . (2018) 85:72–84. doi: 10.1159/000486721
7. Gonzalez-Pons M, Cruz-Correa M. Colorectal cancer biomarkers: where are we now? BioMed Res Int . (2015) 2015:149014. doi: 10.1155/2015/149014
8. Bai Z, Zhou Y, Ye Z, Xiong J, Lan H, Wang F. Tumor-infiltrating lymphocytes in colorectal cancer: the fundamental indication and application on immunotherapy. Front Immunol . (2021) 12:808964. doi: 10.3389/fimmu.2021.808964
9. Guinney J, Dienstmann R, Wang X, de Reyniès A, Schlicker A, Soneson C, et al. The consensus molecular subtypes of colorectal cancer. Nat Med . (2015) 21:1350–6. doi: 10.1038/nm.3967
10. Lech G, Słotwiński R, Słodkowski M, Krasnodębski IW. Colorectal cancer tumour markers and biomarkers: Recent therapeutic advances. World J Gastroenterol . (2016) 22:1745–55. doi: 10.3748/wjg.v22.i5.1745
11. Myer PA, Lee JK, Madison RW, Pradhan K, Newberg JY, Isasi CR, et al. The genomics of colorectal cancer in populations with african and european ancestry. Cancer Discovery . (2022) 12:1282–93. doi: 10.1158/2159-8290.CD-21-0813
12. Lieu CH, Golemis EA, Serebriiskii IG, Newberg J, Hemmerich A, Connelly C, et al. Comprehensive genomic landscapes in early and later onset colorectal cancer. Clin Cancer Res . (2019) 25:5852–8. doi: 10.1158/1078-0432.CCR-19-0899
13. Gao XH, Li J, Liu LJ, Zheng NX, Zheng K, Mei Z, et al. Trends, clinicopathological features, surgical treatment patterns and prognoses of early-onset versus late-onset colorectal cancer: A retrospective cohort study on 34067 patients managed from 2000 to 2021 in a Chinese tertiary center. Int J Surg . (2022) 104:106780. doi: 10.1016/j.ijsu.2022.106780
14. Holowatyj AN, Wen W, Gibbs T, Seagle HM, Keller SR, Edwards DRV, et al. Racial/ethnic and sex differences in somatic cancer gene mutations among patients with early-onset colorectal cancer. Cancer Discovery . (2023) 13:570–9. doi: 10.1158/2159-8290.CD-22-0764
15. Venugopal A, Carethers JM. Epidemiology and biology of early onset colorectal cancer. EXCLI J . (2022) 21:162–82. doi: 10.17179/excli2021-4456
16. Page MJ, McKenzie JE, Bossuyt PM, Boutron I, Hoffmann TC, Mulrow CD, et al. The PRISMA 2020 statement: an updated guideline for reporting systematic reviews. BMJ . (2021) 372:n71. doi: 10.1136/bmj.n71
17. Therkildsen C, Bergmann TK, Henrichsen-Schnack T, Ladelund S, Nilbert M. The predictive value of KRAS, NRAS, BRAF, PIK3CA and PTEN for anti-EGFR treatment in metastatic colorectal cancer: A systematic review and meta-analysis. Acta Oncol . (2014) 53:852–64. doi: 10.3109/0284186X.2014.895036
18. Peeters M, Price TJ, Cervantes A, Sobrero AF, Ducreux M, Hotko Y, et al. Randomized phase III study of panitumumab with fluorouracil, leucovorin, and irinotecan (FOLFIRI) compared with FOLFIRI alone as second-line treatment in patients with metastatic colorectal cancer. J Clin Oncol . (2010) 28:4706–13. doi: 10.1200/JCO.2009.27.6055
19. Formica V, Sera F, Cremolini C, Riondino S, Morelli C, Arkenau HT, et al. KRAS and BRAF mutations in stage II and III colon cancer: A systematic review and meta-analysis. J Natl Cancer Inst . (2022) 114:517–27. doi: 10.1093/jnci/djab190
20. Modest DP, Ricard I, Heinemann V, Hegewisch-Becker S, Schmiegel W, Porschen R, et al. Outcome according to KRAS-, NRAS- and BRAF-mutation as well as KRAS mutation variants: pooled analysis of five randomized trials in metastatic colorectal cancer by the AIO colorectal cancer study group. Ann Oncol . (2016) 27:1746–53. doi: 10.1093/annonc/mdw261
21. De Roock W, Claes B, Bernasconi D, De Schutter J, Biesmans B, Fountzilas G, et al. Effects of KRAS, BRAF, NRAS, and PIK3CA mutations on the efficacy of cetuximab plus chemotherapy in chemotherapy-refractory metastatic colorectal cancer: a retrospective consortium analysis. Lancet Oncol . (2010) 11:753–62. doi: 10.1016/S1470-2045(10)70130-3
22. Schirripa M, Cremolini C, Loupakis F, Morvillo M, Bergamo F, Zoratto F, et al. Role of NRAS mutations as prognostic and predictive markers in metastatic colorectal cancer. Int J Cancer . (2015) 136:83–90. doi: 10.1002/ijc.v136.1
23. Jones JC, Renfro LA, Al-Shamsi HO, Schrock AB, Rankin A, Zhang BY, et al. Non-V600 BRAF mutations define a clinically distinct molecular subtype of metastatic colorectal cancer. J Clin Oncol . (2017) 35:2624–30. doi: 10.1200/JCO.2016.71.4394
24. Yang ZY, Wu XY, Huang YF, Di MY, Zheng DY, Chen JZ, et al. Promising biomarkers for predicting the outcomes of patients with KRAS wild-type metastatic colorectal cancer treated with anti-epidermal growth factor receptor monoclonal antibodies: a systematic review with meta-analysis. Int J Cancer . (2013) 133:1914–25. doi: 10.1002/ijc.v133.8
25. Tan ES, Fan W, Knepper TC, Schell MJ, Sahin IH, Fleming JB, et al. Prognostic and predictive value of PIK3CA mutations in metastatic colorectal cancer. Target Oncol . (2022) 17:483–92. doi: 10.1007/s11523-022-00898-7
26. Wu S, Gan Y, Wang X, Liu J, Li M, Tang Y. PIK3CA mutation is associated with poor survival among patients with metastatic colorectal cancer following anti-EGFR monoclonal antibody therapy: a meta-analysis. J Cancer Res Clin Oncol . (2013) 139:891–900. doi: 10.1007/s00432-013-1400-x
27. Salvatore L, Calegari MA, Loupakis F, Fassan M, Di Stefano B, Bensi M, et al. PTEN in colorectal cancer: shedding light on its role as predictor and target. Cancers (Basel) . (2019) 11:E1765. doi: 10.3390/cancers11111765
CrossRef Full Text | Google Scholar
28. Molinari F, Frattini M. Functions and regulation of the PTEN gene in colorectal cancer. Front Oncol . (2013) 3:326. doi: 10.3389/fonc.2013.00326
29. Liebl MC, Hofmann TG. The role of p53 signaling in colorectal cancer. Cancers (Basel) . (2021) 13:2125. doi: 10.3390/cancers13092125
30. Wang C, Ouyang C, Cho M, Ji J, Sandhu J, Goel A, et al. Wild-type APC is associated with poor survival in metastatic microsatellite stable colorectal cancer. Oncologist . (2021) 26:208–14. doi: 10.1002/onco.13607
31. Jorissen RN, Christie M, Mouradov D, Sakthianandeswaren A, Li S, Love C, et al. Wild-type APC predicts poor prognosis in microsatellite-stable proximal colon cancer. Br J Cancer . (2015) 113:979–88. doi: 10.1038/bjc.2015.296
32. Park DI, Kang MS, Oh SJ, Kim HJ, Cho YK, Sohn CI, et al. HER-2/neu overexpression is an independent prognostic factor in colorectal cancer. Int J Colorectal Dis . (2007) 22:491–7. doi: 10.1007/s00384-006-0192-8
33. Sawada K, Nakamura Y, Yamanaka T, Kuboki Y, Yamaguchi D, Yuki S, et al. Prognostic and predictive value of HER2 amplification in patients with metastatic colorectal cancer. Clin Colorectal Cancer . (2018) 17:198–205. doi: 10.1016/j.clcc.2018.05.006
34. Sartore-Bianchi A, Amatu A, Porcu L, Ghezzi S, Lonardi S, Leone F, et al. HER2 positivity predicts unresponsiveness to EGFR-targeted treatment in metastatic colorectal cancer. Oncologist . (2019) 24:1395–402. doi: 10.1634/theoncologist.2018-0785
35. Marks KM, West NP, Morris E, Quirke P. Clinicopathological, genomic and immunological factors in colorectal cancer prognosis. Br J Surg . (2018) 105:e99–109. doi: 10.1002/bjs.10756
36. Compton CC. Colorectal carcinoma: diagnostic, prognostic, and molecular features. Mod Pathol . (2003) 16:376–88. doi: 10.1097/01.MP.0000062859.46942.93
37. Luo ZW, Zhu MG, Zhang ZQ, Ye FJ, Huang WH, Luo XZ. Increased expression of Ki-67 is a poor prognostic marker for colorectal cancer patients: a meta analysis. BMC Cancer . (2019) 19:123. doi: 10.1186/s12885-019-5324-y
38. Nitsche U, Zimmermann A, Späth C, Müller T, Maak M, Schuster T, et al. Mucinous and signet-ring cell colorectal cancers differ from classical adenocarcinomas in tumor biology and prognosis. Ann Surg . (2013) 258:775–82. doi: 10.1097/SLA.0b013e3182a69f7e
39. An Y, Zhou J, Lin G, Wu H, Cong L, Li Y, et al. Clinicopathological and molecular characteristics of colorectal signet ring cell carcinoma: A review. Pathol Oncol Res . (2021) 27:1609859. doi: 10.3389/pore.2021.1609859
40. Diao Z, Han Y, Chen Y, Zhang R, Li J. The clinical utility of microsatellite instability in colorectal cancer. Crit Rev Oncol Hematol . (2021) 157:103171. doi: 10.1016/j.critrevonc.2020.103171
41. Rhee YY, Kim KJ, Kang GH. CpG island methylator phenotype-high colorectal cancers and their prognostic implications and relationships with the serrated neoplasia pathway. Gut Liver . (2017) 11:38–46. doi: 10.5009/gnl15535
42. Idos GE, Kwok J, Bonthala N, Kysh L, Gruber SB, Qu C. The prognostic implications of tumor infiltrating lymphocytes in colorectal cancer: A systematic review and meta-analysis. Sci Rep . (2020) 10:3360. doi: 10.1038/s41598-020-60255-4
43. Ogino S, Nosho K, Irahara N, Meyerhardt JA, Baba Y, Shima K, et al. Lymphocytic reaction to colorectal cancer is associated with longer survival, independent of lymph node count, microsatellite instability, and CpG island methylator phenotype. Clin Cancer Res . (2009) 15:6412–20. doi: 10.1158/1078-0432.CCR-09-1438
44. Valenzuela G, Canepa J, Simonetti C, Solo de Zaldívar L, Marcelain K, González-Montero J. Consensus molecular subtypes of colorectal cancer in clinical practice: A translational approach. World J Clin Oncol . (2021) 12:1000–8. doi: 10.5306/wjco.v12.i11.1000
45. Herzog R, Álvarez-Pasquin MJ, Díaz C, Del Barrio JL, Estrada JM, Gil Á. Are healthcare workers’ intentions to vaccinate related to their knowledge, beliefs and attitudes? A systematic review. BMC Public Health . (2013) 13:154. doi: 10.1186/1471-2458-13-154
46. Puccini A, Lenz HJ, Marshall JL, Arguello D, Raghavan D, Korn WM, et al. Impact of patient age on molecular alterations of left-sided colorectal tumors. Oncologist . (2019) 24:319–26. doi: 10.1634/theoncologist.2018-0117
47. Tenny S, Hoffman MR. Odds ratio. In: StatPearls . Treasure Island (FL): StatPearls Publishing. (2024) Available at: http://www.ncbi.nlm.nih.gov/books/NBK431098/ .
Google Scholar
48. Riley RD, Higgins JPT, Deeks JJ. Interpretation of random effects meta-analyses. BMJ . (2011) 342:d549. doi: 10.1136/bmj.d549
49. Wang MJ, Ping J, Li Y, Adell G, Arbman G, Nodin B, et al. The prognostic factors and multiple biomarkers in young patients with colorectal cancer. Sci Rep . (2015) 5:10645. doi: 10.1038/srep10645
50. Du M, Gu D, Xin J, Peters U, Song M, Cai G, et al. Integrated multi-omics approach to distinct molecular characterization and classification of early-onset colorectal cancer. Cell Rep Med . (2023) 4:100974. doi: 10.1016/j.xcrm.2023.100974
51. Ugai T, Väyrynen JP, Lau MC, Borowsky J, Akimoto N, Väyrynen SA, et al. Immune cell profiles in the tumor microenvironment of early-onset, intermediate-onset, and later-onset colorectal cancer. Cancer Immunol Immunother . (2022) 71:933–42. doi: 10.1007/s00262-021-03056-6
52. Gardner IH, Siddharthan R, Watson K, Dewey E, Ruhl R, Khou S, et al. A distinct innate immune signature of early onset colorectal cancer. Immunohorizons . (2021) 5:489–99. doi: 10.4049/immunohorizons.2000092
53. Irabor DO, Oluwasola OA, Ogunbiyi OJ, Ogun OG, Okolo CA, Melas M, et al. Microsatellite instability is common in colorectal cancer in native Nigerians. Anticancer Res . (2017) 37:2649–54. doi: 10.21873/anticanres
54. Li GM, Xiao GZ, Qin PF, Wan XY, Fu YJ, Zheng YH, et al. Single-cell RNA sequencing reveals heterogeneity in the tumor microenvironment between young-onset and old-onset colorectal cancer. Biomolecules . (2022) 12:1860. doi: 10.3390/biom12121860
55. Pilozzi E, Maresca C, Duranti E, Giustiniani MC, Catalanotto C, Lucarelli M, et al. Left-sided early-onset vs late-onset colorectal carcinoma: histologic, clinical, and molecular differences. Am J Clin Pathol . (2015) 143:374–84. doi: 10.1309/AJCPNOC55IOLXFUD
56. Andric F, Al-Fairouzi A, Wettergren Y, Szeponik L, Bexe-Lindskog E, Cusack JC, et al. Immune microenvironment in sporadic early-onset versus average-onset colorectal cancer. Cancers (Basel) . (2023) 15:1457. doi: 10.3390/cancers15051457
57. Lu C, Zhang X, Schardey J, Wirth U, Heinrich K, Massiminio L, et al. Molecular characteristics of microsatellite stable early-onset colorectal cancer as predictors of prognosis and immunotherapeutic response. NPJ Precis Oncol . (2023) 7:63. doi: 10.1038/s41698-023-00414-8
58. Willauer AN, Liu Y, Pereira AAL, Lam M, Morris JS, Raghav KPS, et al. Clinical and molecular characterization of early-onset colorectal cancer. Cancer . (2019) 125:2002–10. doi: 10.1002/cncr.31994
59. Ha YJ, Shin YJ, Tak KH, Park JL, Kim JH, Lee JL, et al. Reduced expression of alanyl aminopeptidase is a robust biomarker of non-familial adenomatous polyposis and non-hereditary nonpolyposis colorectal cancer syndrome early-onset colorectal cancer. Cancer Med . (2023) 12:10091–104. doi: 10.1002/cam4.5675
60. Yang J, Zhao Y, Yuan R, Wang Y, Wang S, Chang Z, et al. Identifying individualized prognostic signature and unraveling the molecular mechanism of recurrence in early-onset colorectal cancer. Eur J Med Res . (2023) 28:533. doi: 10.1186/s40001-023-01491-y
61. Furuhashi S, Bustos MA, Mizuno S, Ryu S, Naeini Y, Bilchik AJ, et al. Spatial profiling of cancer-associated fibroblasts of sporadic early onset colon cancer microenvironment. NPJ Precis Oncol . (2023) 7:118. doi: 10.1038/s41698-023-00474-w
62. Chang SH, Patel N, Du M, Liang PS. Trends in early-onset vs late-onset colorectal cancer incidence by race/ethnicity in the United States cancer statistics database. Clin Gastroenterol Hepatol . (2022) 20:e1365–77. doi: 10.1016/j.cgh.2021.07.035
63. Wang R, Wang MJ, Ping J. Clinicopathological features and survival outcomes of colorectal cancer in young versus elderly: A population-based cohort study of SEER 9 registries data (1988-2011). Med (Baltimore) . (2015) 94:e1402. doi: 10.1097/MD.0000000000001402
64. McClelland PHT, Liu T, Ozuner G. Early-onset colorectal cancer in patients under 50 years of age: demographics, disease characteristics, and survival. Clin Colorectal Cancer . (2022) 21:e135–44. doi: 10.1016/j.clcc.2021.11.003
65. Saraiva MR, Rosa I, Claro I. Early-onset colorectal cancer: A review of current knowledge. World J Gastroenterol . (2023) 29:1289–303. doi: 10.3748/wjg.v29.i8.1289
66. Cavestro GM, Mannucci A, Balaguer F, Hampel H, Kupfer SS, Repici A, et al. Delphi initiative for early-onset colorectal cancer (DIRECt) international management guidelines. Clin Gastroenterol Hepatol . (2023) 21:581–603. doi: 10.1016/j.cgh.2022.12.006
67. Kneuertz PJ, Chang GJ, Hu CY, Rodriguez-Bigas MA, Eng C, Vilar E, et al. Overtreatment of young adults with colon cancer: more intense treatments with unmatched survival gains. JAMA Surg . (2015) 150:402–9. doi: 10.1001/jamasurg.2014.3572
68. Abdelsattar ZM, Wong SL, Regenbogen SE, Jomaa DM, Hardiman KM, Hendren S. Colorectal cancer outcomes and treatment patterns in patients too young for average-risk screening. Cancer . (2016) 122:929–34. doi: 10.1002/cncr.29716
69. Kanter K, Fish M, Mauri G, Horick NK, Allen JN, Blaszkowsky LS, et al. Care patterns and overall survival in patients with early-onset metastatic colorectal cancer. JCO Oncol Pract . (2021) 17:e1846–55. doi: 10.1200/OP.20.01010
70. NCCN. Guidelines Detail (2023). Available online at: https://www.nccn.org/guidelines/guidelines-detail .
71. Afrăsânie VA, Marinca MV, Alexa-Stratulat T, Gafton B, Păduraru M, Adavidoaiei AM, et al. KRAS, NRAS, BRAF, HER2 and microsatellite instability in metastatic colorectal cancer - practical implications for the clinician. Radiol Oncol . (2019) 53:265–74. doi: 10.2478/raon-2019-0033
72. Taieb J, Zaanan A, Le Malicot K, Julié C, Blons H, Mineur L, et al. Prognostic effect of BRAF and KRAS mutations in patients with stage III colon cancer treated with leucovorin, fluorouracil, and oxaliplatin with or without cetuximab: A post hoc analysis of the PETACC-8 trial. JAMA Oncol . (2016) 2:643–53. doi: 10.1001/jamaoncol.2015.5225
73. Jácome AA, Vreeland TJ, Johnson B, Kawaguchi Y, Wei SH, Nancy You Y, et al. The prognostic impact of RAS on overall survival following liver resection in early versus late-onset colorectal cancer patients. Br J Cancer . (2021) 124:797–804. doi: 10.1038/s41416-020-01169-w
74. Aljehani MA, Bien J, Lee JSH, Fisher GA, Lin AY. KRAS sequence variation as prognostic marker in patients with young- vs late-onset colorectal cancer. JAMA Netw Open . (2023) 6:e2345801. doi: 10.1001/jamanetworkopen.2023.45801
75. Khan SA, Morris M, Idrees K, Gimbel MI, Rosenberg S, Zeng Z, et al. Colorectal cancer in the very young: a comparative study of tumor markers, pathology and survival in early onset and adult onset patients. J Pediatr Surg . (2016) 51:1812–7. doi: 10.1016/j.jpedsurg.2016.07.015
76. Irahara N, Baba Y, Nosho K, Shima K, Yan L, Dias-Santagata D, et al. NRAS mutations are rare in colorectal cancer. Diagn Mol Pathol . (2010) 19:157–63. doi: 10.1097/PDM.0b013e3181c93fd1
77. Capper D, Voigt A, Bozukova G, Ahadova A, Kickingereder P, von Deimling A, et al. BRAF V600E-specific immunohistochemistry for the exclusion of Lynch syndrome in MSI-H colorectal cancer. Int J Cancer . (2013) 133:1624–30. doi: 10.1002/ijc.28183
78. Danielsen SA, Eide PW, Nesbakken A, Guren T, Leithe E, Lothe RA. Portrait of the PI3K/AKT pathway in colorectal cancer. Biochim Biophys Acta . (2015) 1855:104–21. doi: 10.1016/j.bbcan.2014.09.008
79. Li QH, Wang YZ, Tu J, Liu CW, Yuan YJ, Lin R, et al. Anti-EGFR therapy in metastatic colorectal cancer: mechanisms and potential regimens of drug resistance. Gastroenterol Rep (Oxf) . (2020) 8:179–91. doi: 10.1093/gastro/goaa026
80. Nakayama M, Oshima M. Mutant p53 in colon cancer. J Mol Cell Biol . (2019) 11:267–76. doi: 10.1093/jmcb/mjy075
81. Michel M, Kaps L, Maderer A, Galle PR, Moehler M. The role of p53 dysfunction in colorectal cancer and its implication for therapy. Cancers (Basel) . (2021) 13:2296. doi: 10.3390/cancers13102296
82. Aghabozorgi AS, Bahreyni A, Soleimani A, Bahrami A, Khazaei M, Ferns GA, et al. Role of adenomatous polyposis coli (APC) gene mutations in the pathogenesis of colorectal cancer; current status and perspectives. Biochimie . (2019) 157:64–71. doi: 10.1016/j.biochi.2018.11.003
83. Li J, Ma X, Chakravarti D, Shalapour S, DePinho RA. Genetic and biological hallmarks of colorectal cancer. Genes Dev . (2021) 35:787–820. doi: 10.1101/gad.348226.120
84. Sinicrope FA. Lynch syndrome-associated colorectal cancer. N Engl J Med . (2018) 379:764–73. doi: 10.1056/NEJMcp1714533
85. McRonald FE, Pethick J, Santaniello F, Shand B, Tyson A, Tulloch O, et al. Identification of people with Lynch syndrome from those presenting with colorectal cancer in England: baseline analysis of the diagnostic pathway. Eur J Hum Genet . (2024). doi: 10.1038/s41431-024-01550-w
86. Mezzapesa M, Losurdo G, Celiberto F, Rizzi S, d’Amati A, Piscitelli D, et al. Serrated colorectal lesions: an up-to-date review from histological pattern to molecular pathogenesis. Int J Mol Sci . (2022) 23:4461. doi: 10.3390/ijms23084461
87. Barresi V, Reggiani Bonetti L, Ieni A, Caruso RA, Tuccari G. Histological grading in colorectal cancer: new insights and perspectives. Histol Histopathol . (2015) 30:1059–67. doi: 10.14670/HH-11-633
88. Fadel MG, Malietzis G, Constantinides V, Pellino G, Tekkis P, Kontovounisios C. Clinicopathological factors and survival outcomes of signet-ring cell and mucinous carcinoma versus adenocarcinoma of the colon and rectum: a systematic review and meta-analysis. Discovery Oncol . (2021) 12:5. doi: 10.1007/s12672-021-00398-6
89. Luo C, Cen S, Ding G, Wu W. Mucinous colorectal adenocarcinoma: clinical pathology and treatment options. Cancer Commun (Lond) . (2019) 39:13. doi: 10.1186/s40880-019-0361-0
90. Gabriel E, Attwood K, Al-Sukhni E, Erwin D, Boland P, Nurkin S. Age-related rates of colorectal cancer and the factors associated with overall survival. J Gastrointest Oncol . (2018) 9:96–110. doi: 10.21037/jgo
91. Ding X, Yang X, Wu D, Huang Y, Dai Y, Li J, et al. Nomogram predicting the cancer-specific survival of early-onset colorectal cancer patients with synchronous liver metastasis: a population-based study. Int J Colorectal Dis . (2022) 37:1309–19. doi: 10.1007/s00384-022-04175-x
92. Chen Y, He L, Lu X, Tang Y, Luo G, Chen Y, et al. Causes of death among early-onset colorectal cancer population in the United States: a large population-based study. Front Oncol . (2023) 13:1094493. doi: 10.3389/fonc.2023.1094493
93. Benesch MGK, Mathieson A, O’Brien SBL. Effects of tumor localization, age, and stage on the outcomes of gastric and colorectal signet ring cell adenocarcinomas. Cancers (Basel) . (2023) 15:714. doi: 10.3390/cancers15030714
94. De Renzi G, Gaballo G, Gazzaniga P, Nicolazzo C. Molecular biomarkers according to primary tumor location in colorectal cancer: current standard and new insights. Oncology . (2021) 99:135–43. doi: 10.1159/000510944
95. Cheng E, Blackburn HN, Ng K, Spiegelman D, Irwin ML, Ma X, et al. Analysis of survival among adults with early-onset colorectal cancer in the national cancer database. JAMA Netw Open . (2021) 4:e2112539. doi: 10.1001/jamanetworkopen.2021.12539
96. Charlton ME, Kahl AR, Greenbaum AA, Karlitz JJ, Lin C, Lynch CF, et al. KRAS testing, tumor location, and survival in patients with stage IV colorectal cancer: SEER 2010-2013. J Natl Compr Canc Netw . (2017) 15:1484–93. doi: 10.6004/jnccn.2017.7011
97. Xie MZ, Li JL, Cai ZM, Li KZ, Hu BL. Impact of primary colorectal Cancer location on the KRAS status and its prognostic value. BMC Gastroenterol . (2019) 19:46. doi: 10.1186/s12876-019-0965-5
98. Lee MS, Menter DG, Kopetz S. Right versus left colon cancer biology: integrating the consensus molecular subtypes. J Natl Compr Canc Netw . (2017) 15:411–9. doi: 10.6004/jnccn.2017.0038
99. Foppa C, Tamburello S, Maroli A, Carvello M, Poliani L, Laghi L, et al. Early age of onset is an independent predictor for worse disease-free survival in sporadic rectal cancer patients. A comparative analysis of 980 consecutive patients. Eur J Surg Oncol . (2022) 48:857–63. doi: 10.1016/j.ejso.2021.10.021
100. Laskar RS, Ghosh SK, Talukdar FR. Rectal cancer profiling identifies distinct subtypes in India based on age at onset, genetic, epigenetic and clinicopathological characteristics. Mol Carcinog . (2015) 54:1786–95. doi: 10.1002/mc.22250
101. Perea J, García JL, Corchete L, Tapial S, Olmedillas-López S, Vivas A, et al. A clinico-pathological and molecular analysis reveals differences between solitary (early and late-onset) and synchronous rectal cancer. Sci Rep . (2021) 11:2202. doi: 10.1038/s41598-020-79118-z
102. Spolverato G, Fassan M, Scarpa M, Stepanyan A, De Simoni O, Scognamiglio F, et al. IMMUNOREACT 6: weak immune surveillance characterizes early-onset rectal cancer. Br J Surg . (2023) 110:1490–501. doi: 10.1093/bjs/znad219
103. Ugai T, Haruki K, Harrison TA, Cao Y, Qu C, Chan AT, et al. Molecular characteristics of early-onset colorectal cancer according to detailed anatomical locations: comparison with later-onset cases. Am J Gastroenterol . (2023) 118:712–26. doi: 10.14309/ajg.0000000000002171
104. Baran B, Mert Ozupek N, Yerli Tetik N, Acar E, Bekcioglu O, Baskin Y. Difference between left-sided and right-sided colorectal cancer: A focused review of literature. Gastroenterol Res . (2018) 11:264–73. doi: 10.14740/gr1062w
105. Galon J, Costes A, Sanchez-Cabo F, Kirilovsky A, Mlecnik B, Lagorce-Pagès C, et al. Type, density, and location of immune cells within human colorectal tumors predict clinical outcome. Science . (2006) 313:1960–4. doi: 10.1126/science.1129139
106. Angell HK, Bruni D, Barrett JC, Herbst R, Galon J. The immunoscore: colon cancer and beyond. Clin Cancer Res . (2020) 26:332–9. doi: 10.1158/1078-0432.CCR-18-1851
107. Ten Hoorn S, de Back TR, Sommeijer DW, Vermeulen L. Clinical value of consensus molecular subtypes in colorectal cancer: A systematic review and meta-analysis. J Natl Cancer Inst . (2022) 114:503–16. doi: 10.1093/jnci/djab106
Keywords: colorectal cancer, colon cancer, rectal cancer, early-onset, oncogenes, prognosis, molecular characteristics
Citation: Lawler T, Parlato L and Warren Andersen S (2024) The histological and molecular characteristics of early-onset colorectal cancer: a systematic review and meta-analysis. Front. Oncol. 14:1349572. doi: 10.3389/fonc.2024.1349572
Received: 04 December 2023; Accepted: 16 April 2024; Published: 26 April 2024.
Reviewed by:
Copyright © 2024 Lawler, Parlato and Warren Andersen. This is an open-access article distributed under the terms of the Creative Commons Attribution License (CC BY) . The use, distribution or reproduction in other forums is permitted, provided the original author(s) and the copyright owner(s) are credited and that the original publication in this journal is cited, in accordance with accepted academic practice. No use, distribution or reproduction is permitted which does not comply with these terms.
*Correspondence: Shaneda Warren Andersen, [email protected]
Disclaimer: All claims expressed in this article are solely those of the authors and do not necessarily represent those of their affiliated organizations, or those of the publisher, the editors and the reviewers. Any product that may be evaluated in this article or claim that may be made by its manufacturer is not guaranteed or endorsed by the publisher.
- Open access
- Published: 26 April 2024
SF3B3-regulated mTOR alternative splicing promotes colorectal cancer progression and metastasis
- Tong Xu 1 ,
- Xichuan Li 2 ,
- Wennan Zhao 1 ,
- Xue Wang 3 ,
- Leixin Jin 4 ,
- Zhiqiang Feng 4 ,
- Huixiang Li 1 ,
- Mingzhe Zhang 1 ,
- Yiqing Tian 1 ,
- Yuan Yue 2 ,
- Xintong Dai 5 ,
- Changliang Shan 5 ,
- Weihua Zhang 6 ,
- Chunze Zhang 4 &
- Youcai Zhang ORCID: orcid.org/0000-0001-9919-6442 1
Journal of Experimental & Clinical Cancer Research volume 43 , Article number: 126 ( 2024 ) Cite this article
413 Accesses
1 Altmetric
Metrics details
Aberrant alternative splicing (AS) is a pervasive event during colorectal cancer (CRC) development. SF3B3 is a splicing factor component of U2 small nuclear ribonucleoproteins which are crucial for early stages of spliceosome assembly. The role of SF3B3 in CRC remains unknown.
SF3B3 expression in human CRCs was analyzed using publicly available CRC datasets, immunohistochemistry, qRT-PCR, and western blot. RNA-seq, RNA immunoprecipitation, and lipidomics were performed in SF3B3 knockdown or overexpressing CRC cell lines. CRC cell xenografts, patient-derived xenografts, patient-derived organoids, and orthotopic metastasis mouse models were utilized to determine the in vivo role of SF3B3 in CRC progression and metastasis.
SF3B3 was upregulated in CRC samples and associated with poor survival. Inhibition of SF3B3 by RNA silencing suppressed the proliferation and metastasis of CRC cells in vitro and in vivo, characterized by mitochondria injury, increased reactive oxygen species (ROS), and apoptosis. Mechanistically, silencing of SF3B3 increased mTOR exon-skipped splicing, leading to the suppression of lipogenesis via mTOR-SREBF1-FASN signaling. The combination of SF3B3 shRNAs and mTOR inhibitors showed synergistic antitumor activity in patient-derived CRC organoids and xenografts. Importantly, we identified SF3B3 as a critical regulator of mTOR splicing and autophagy in multiple cancers.
Conclusions
Our findings revealed that SF3B3 promoted CRC progression and metastasis by regulating mTOR alternative splicing and SREBF1-FASN-mediated lipogenesis, providing strong evidence to support SF3B3 as a druggable target for CRC therapy.
Colorectal cancer (CRC) ranks the second most commonly diagnosed cancer and the third-highest cause of cancer-related deaths worldwide [ 1 ]. Remarkably, at the time of diagnosis, 10–30% of CRC patients present with distant metastasis, with approximately 50% developing liver metastasis, the leading cause of mortality in CRC patients [ 2 ]. While surgical resection is considered the sole potentially curative therapy for CRC, many advanced CRC patients require chemotherapy. Nevertheless, only a small percentage of CRC patients with metastasis manage to survive for more than 5 years even after chemotherapy or surgery. The challenge in achieving effective CRC therapy stems largely from a limited understanding of the fundamental pathological mechanisms underlying CRC tumorigenesis and metastasis.
More than 90% of mammalian genes undergo alternative splicing (AS), a process through which several mRNA variants can be generated from a single gene. AS is executed by a sophisticated spliceosome machinery, which recognizes different splice sites and catalyzes two fundamental transesterification reactions. The core spliceosome comprises five small nuclear ribonucleoprotein (snRNPs) complexes (U1, U2, U4, U5, and U6 snRNP) and over 200 related proteins. Alterations in either cis-acting RNA sequence elements or trans-acting regulation splicing factors result in splicing dysregulation, which underlies various human diseases, including cancer [ 3 ]. Accumulating evidence suggests that aberrant AS is associated with CRC proliferation, invasion, apoptosis, angiogenesis, and drug-resistance [ 4 , 5 , 6 ].
The mammalian or mechanistic target of rapamycin (mTOR) is a serine/threonine kinase involved in regulating autophagy, metabolism, survival, and the immune response. Hyperactivation of the mTOR pathway contributes to tumor initiation and progression [ 7 , 8 ]. Targeting mTOR has emerged as an effective therapeutic approach for various cancers, including CRC [ 9 ]. While several mTOR splicing variants are documented in database, only two functional human mTOR isoforms have been identified, namely mTORα and mTORβ [ 10 ]. To date, the splicing mechanism for mTORβ as well as the role of mTOR splicing in tumor development remain elusive.
The SF3b complex is an intrinsic component of the U2 snRNP and plays a crucial role in recognizing the branch point sequence (BPS) during early stages of spliceosome assembly [ 11 ]. The SF3b complex comprises seven proteins (SF3B1, SF3B2, SF3B3, SF3B4, SF3B5, SF3B6, and SF3B7), each demonstrated to possess distinct structures and functions. SF3B1 and SF3B7 (also known as PHD finger protein 5A, PHF5A) have been extensively investigated for their functional roles in multiple cancers, including CRC [ 4 , 12 , 13 , 14 , 15 ]. However, whether other SF3b components play a role in CRC remains unknown. In this study, we systematically investigated the role of SF3B3 in regulating AS events and gene expression in CRC cells. We reported that SF3B3 overexpression was common in CRC patients, and was clinically related with CRC prognosis. SF3B3 facilitated the proliferation and metastasis of CRC cells in vitro and in vivo. Mechanistically, SF3B3 promoted lipogenesis by regulating mTOR-SREBF1-FASN signaling. Importantly, we identified SF3B3 as a critical regulator of mTOR splicing and autophagy in multiple cancers, including CRC.
Materials and methods
Animal studies.
BALB/C nude mice (male and female, 4–5 weeks of age) were obtained from Beijing Vital River Laboratory Animal Technology Co., Ltd. (Beijing, China). NSG mice (female, 6–8 weeks of age) were obtained from Gem Pharmatech (Jiangsu, China). The protocol for mouse housing and usage was approved by the Laboratory Animal Ethics Committee of Tianjin Haihe hospital (Tianjin, China).
Cell culture
The human CRC cell lines LoVo, HT29, SW480 and other cancer cell lines were obtained from National Infrastructure of Cell Line Resource (Beijing, China). The HEK293T cell line was acquired from Cell Resource Center of Shanghai Institutes for Biological Sciences (Shanghai, China). LoVo, SW480, Hela, MCF7, Huh7, and HEK293T cells were cultured in Dulbecco’s modified Eagle’s medium (DMEM) supplemented with 10% fetal bovine serum (Cell-Box, AUS-01S-02) and 1% penicillin/streptomycin. HT29 cells were maintained in RPMI-1640 medium supplemented with 10% fetal bovine serum and 1% penicillin/streptomycin. All cells were incubated in a humidified incubator at 37 °C with 5% CO 2 .
Human samples and ethical statement
A total of 127 paired human CRC and adjacent noncancerous tissues were obtained from Tianjin Union Medical Center (Tianjin, China). Among these, 98 paired human CRC and adjacent noncancerous tissues were utilized for tissue microarray, while 25 paired tissues were used for qRT-PCR and western blot analysis. Three patient CRC tissues obtained at the time of surgery were used for constructing PDX models. One patient CRC tissue was used for construction of CRC organoids. The study adhered to the recommendations outlined in the Requirements of the Ethical Review System of Biomedical Research Involving Human by Tianjin Union Medical Center Ethics Committee. All subjects were given a written informed consent in accordance with the Declaration of Helsinki.
A more detailed description of materials and methods is provided in the Supplemental Materials.
SF3B3 is overexpressed in CRC
The mRNA expression of SF3b components ( SF3B1-SF3B7 ) was analyzed using The Cancer Genome Atlas-colonic adenocarcinoma (TCGA-COAD) and rectal adenocarcinoma (TCGA-READ) datasets. Consistent with a previous study [ 14 ], we observed significantly elevated mRNA levels of both SF3B3 and SF3B7 in CRCs ( n = 383) compared to surrounding normal tissues ( n = 51) (Fig. 1 A and Fig. S 1 A). Notably, SF3B3 mRNA levels were substantially higher than SF3B7 mRNA levels in CRC tissues (Fig. S 1 B). Furthermore, only SF3B3 mRNA expression was significantly upregulated in CRC tissues when normal colon tissues from the Genotype-Tissue Expression (GTEx) datasets were integrated with TCGA datasets (Fig. S 1 C). SF3B3 mRNA levels were also higher in CRC tumors than their matched-paired normal tissues according to the analysis of samples from TCGA datasets and two recently published Gene Expression Omnibus (GEO) datasets (Fig. 1 B).
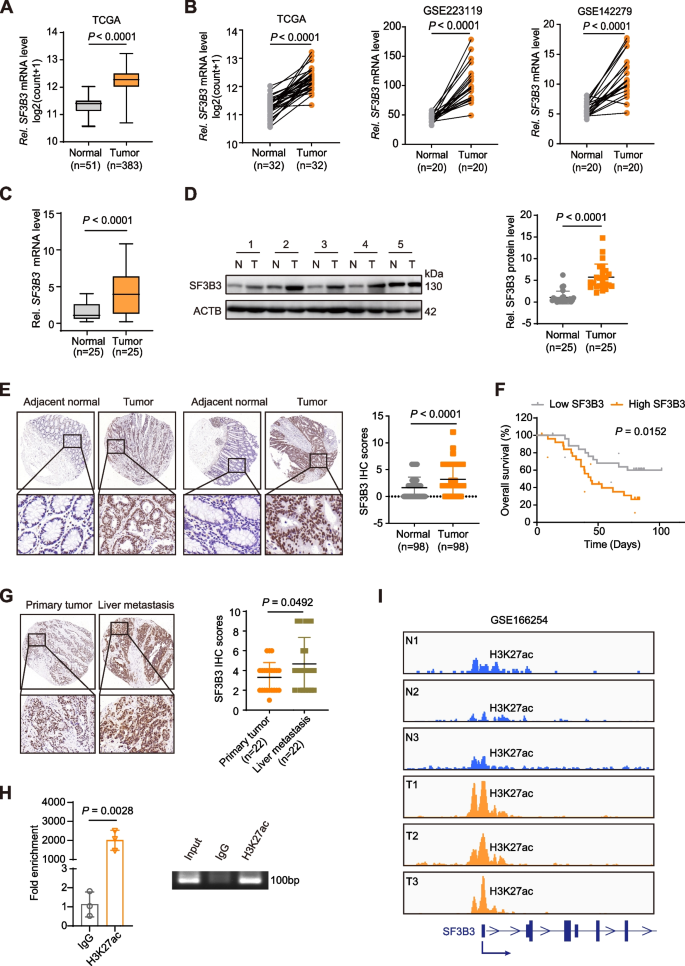
SF3B3 expression is upregulated in human CRC. A SF3B3 transcript levels in normal and CRC tissues. The data were obtained from TCGA-COAD and TCGA-READ datasets in Xena ( http://xena.ucsc.edu/ ). B Relative SF3B3 mRNA levels in CRC and pair matched adjacent normal tissues. The data were obtained from TCGA-COAD and TCGA-READ datasets in Xena, as well as GEO datasets. C Relative SF3B3 mRNA levels in paired CRC and adjacent normal tissues. The mRNA expression was determined by qRT-PCR. D Western blot analysis of SF3B3 protein in paired CRC and adjacent normal tissues. E Representative IHC staining images of SF3B3 protein and statistical analysis of SF3B3 IHC scores in CRC and adjacent normal tissues. The staining extent was scored on a scale of 0 − 4. The staining intensity was scored at 0–3 (3 is the highest positivity). The final IHC score was generated by multiplying the score of staining extent with the score of staining intensity. The maximum score is 12. F Kaplan–Meier analysis of overall survival from CRC patients with high and low SF3B3 protein levels ( n = 29). G Representative IHC staining images of SF3B3 protein and statistical analysis of SF3B3 IHC scores in primary CRC and their corresponding liver metastasis tissues. The final IHC score was generated by multiplying the score of staining extent with the score of staining intensity. H ChIP-PCR analysis of the enrichment of H3K27ac in SF3B3 promoter region relative to IgG in LoVo cells. The immunoprecipitated DNA fragments by anti-H3K27ac and IgG were purified and analyzed by PCR with primers specific for SF3B3 promoter. I Genome browser view of H3K27ac ChIP-seq data in SF3B3 promoter region from CRC patient tissues. Data are shown as mean ± SD
We then explored the correlation of SF3B3 expression with other genes using TCGA datasets. A total of 1574 genes showed positive co-expression with SF3B3 , while 993 genes exhibited negative co-expression with SF3B3 (| r |> 0.2, p < 0.05) (Tables S 1 and S2). KEGG pathway enrichment analysis revealed that SF3B3 positively co-expressed genes were most significantly enriched in the “spliceosome” pathway, whereas SF3B3 negatively co-expressed genes were predominantly enriched in the “metabolic” pathway (Fig. S 1 D). Notably, SF3B3 expression demonstrated a positive correlation with MKI67 , which encodes a proliferation marker for tumor cells (Fig. S 1 E).
To validate the dataset analysis results, we quantified SF3B3 expression using qRT-PCR and western blot in 25 paired human CRC and adjacent normal tissues (Fig. 1 C-D). Additionally, we performed immunohistochemical (IHC) analysis of SF3B3 in a cohort of 98 paired CRC and matched adjacent normal tissues (Fig. 1 E). All these data supported the upregulation of SF3B3 expression in CRC tissues. Kaplan–Meier analysis revealed that CRC patients with high SF3B3 levels had significantly reduced overall survival compared to those with low SF3B3 levels (Fig. 1 F). Interestingly, SF3B3 protein levels in liver metastatic lesions were significantly higher than those in the matched primary CRC tissues ( n = 22) (Fig. 1 G). These clinical data strongly associate SF3B3 expression with CRC metabolism, RNA splicing, proliferation, progression and prognosis.
As epigenetic modification was shown to be involved in the regulation of SF3B7 expression [ 14 ], we investigated whether SF3B3 expression was also epigenetically regulated. The Cistrome DB Toolkit ( http://dbtoolkit.cistrome.org ) was employed to analyze potential epigenetic factors for SF3B3 [ 16 ]. Among all histone modifications, histone H3-lysine-27 (H3K27ac) exhibited the highest regulatory potential score across all chromatin immunoprecipitation sequencing (ChIP-seq) samples (Fig. S 1 F). H3K27ac was found to be notably enriched in the promoter of SF3B3 , as determined using the UCSC Genome Bioinformatics Site ( http://genome.ucsc.edu/ ) (Fig. S 1 G). ChIP-seq data analysis in CRC cells also identified H3K27ac occupancy in the promoter region of SF3B3 (Fig. S 1 H). Furthermore, we confirmed this occupancy through ChIP-PCR in CRC cells (Fig. 1 H). Treatment with curcumin, an inhibitor of histone acetylation, was able to downregulate the expression of SF3B3 , as well as a known H3K27ac-targeted gene FOXM1 (Fig. S 1 I). ChIP-seq data analysis of CRC patient tissues (GEO: GSE166254) revealed that the H3K27ac peak in SF3B3 promoter region was significantly higher in tumors than in normal tissues (Fig. 1 I). These findings suggest that the upregulation of SF3B3 in CRC tissues is at least partially attributed to promoter histone acetylation.
SF3B3 promotes CRC proliferation, migration and invasion in vitro
The functional roles of SF3B3 were determined in CRC cell lines. We found that SF3B3 mRNA and protein levels were generally higher in CRC cells compared to normal human mucosal epithelial cells (Fig. S 2 A). LoVo and HT29 cells with high SF3B3 expression were selected for knockdown experiments. Two SF3B3 specific siRNAs were identified, and their knockdown effects were validated at both mRNA and protein levels (Fig. S 2 B). Both SF3B3 siRNAs significantly suppressed cell viability (Fig. 2 A, Fig. S 2 C) and colony formation (Fig. 2 B, Fig. S 2 D) of LoVo and HT29 cells, which were largely rescued by SF3B3 re-expression. Conversely, SF3B3 overexpression promoted cell growth and colony formation of SW480 cells that had low endogenous SF3B3 expression (Fig. S 2 B, E).
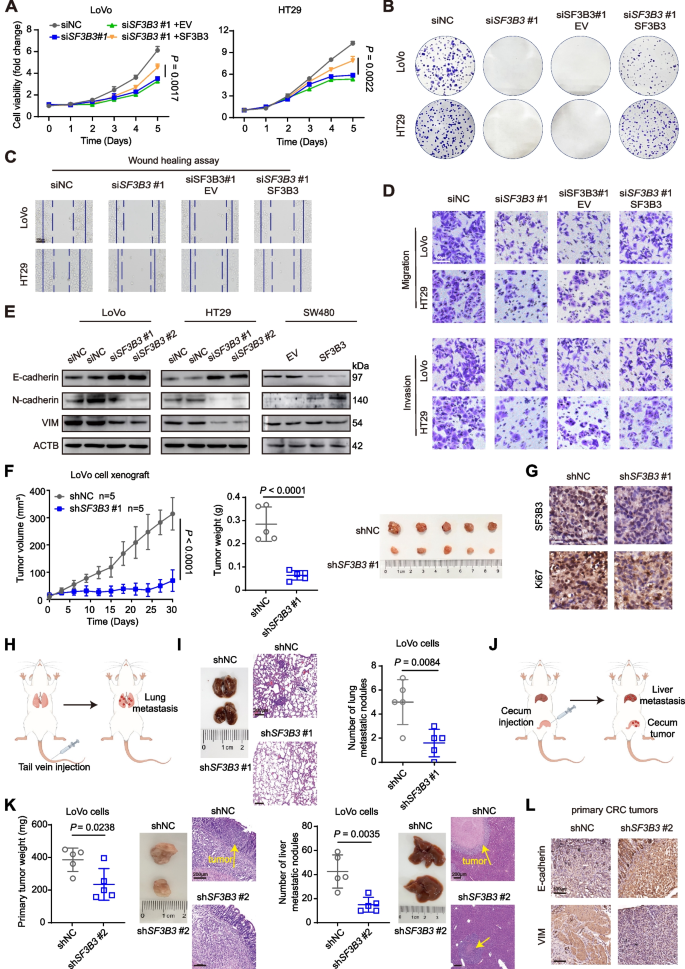
SF3B3 promotes the proliferation and metastasis of CRC in Vitro and Vivo . A Growth curves and ( B ) colony formation of LoVo and HT29 cells after SF3B3 knockdown by siRNAs (siNC vs si SF3B3 ), or after SF3B3 knockdown by siRNAs for 12 h followed with re-expression of SF3B3 (si SF3B3 + EV vs si SF3B3 + SF3B3) (empty vector, EV; SF3B3 overexpressing plasmid, SF3B3). Cell viability was determined at different time points and colony formation assay was measured after 2 weeks. C Wound healing assays and ( D ) Transwell assays of LoVo and HT29 cells to investigate the effects of SF3B3 knockdown (siNC vs si SF3B3 ) or re-expression (si SF3B3 + EV vs si SF3B3 + SF3B3) on cell migration and invasion abilities. Scale bars, 100 μm. E Representative western blots of EMT-related proteins in SF3B3 -knockdown or SF3B3 -overexpressing CRC cells. F Tumor growth, tumor weights, and tumor images of xenografts in nude mice. LoVo cells were infected with shNC or sh SF3B3 #1 lentivirus to obtain the stably cell clones, which were subcutaneously injected into flank region of each nude mouse. G Representative IHC images of SF3B3 and Ki67 proteins in xenografts. Scale bars, 25 μm. H Schematic design of the CRC lung metastasis mouse model. I Representative images of lung, H&E staining of lung tissues derived from mice after tail vein injection with stably SF3B3 -knockdown LoVo cells (LoVo-sh SF3B3 #1), and statistical analysis of lung metastatic nodules. Scale bars, 200 μm. J Schematic design of the CRC liver metastasis mouse model. K Statistical analysis of primary CRC tumor weights and liver metastatic nodules, as well as representative H&E staining. Stably SF3B3 -knockdown LoVo cells were constructed using sh SF3B3 #2 lentivirus. Scale bars, 200 μm. L Representative IHC images of E-cadherin and VIM in primary CRC tumors from mice orthotopically injected with SF3B3 -knockdown LoVo cells (LoVo-sh SF3B3 #2). Scale bars, 100 μm. Data are presented as mean ± SD
We then determined the impact of SF3B3 on the invasive and migratory ability of CRC cells using Wound healing scratch assays and Transwell assays. SF3B3 siRNAs markedly suppressed the migration and invasion capacity of LoVo and HT29 cells, which could be rescued by SF3B3 re-expression (Fig. 2 C-D, Fig. S 2 F-H). Epithelial-mesenchymal transition (EMT) is an important event during cancer invasion and metastasis. Western blot and immunofluorescence staining analyses revealed that SF3B3 knockdown enhanced the level of the epithelial marker E-cadherin, while reducing the levels of the mesenchymal markers vimentin (VIM) and N-cadherin (Fig. 2 E, Fig. S 2 I). Conversely, SF3B3 overexpression exerted the opposite effects on EMT markers. These findings indicate that SF3B3 promotes the proliferation and metastasis of CRC cells in vitro.
Silencing of SF3B3 impedes CRC proliferation and metastasis in vivo
To investigate the in vivo function of SF3B3, we utilized a lentivirus-mediated shRNA system to construct stably SF3B3 -knockdown CRC cells (LoVo-sh SF3B3 #1, LoVo-sh SF3B3 #2 cells, HT29-sh SF3B3 #2 cells) for mouse CRC models (Fig. S 3 A-B). Firstly, LoVo-sh SF3B3 #1 and control (LoVo-shNC) cells were subcutaneously injected into nude mice for xenograft tumor formation. Compared to controls, the growth of LoVo-sh SF3B3 #1 xenografts was significantly suppressed with no significant difference in mouse body weights (Fig. 2 F, Fig. S 3 C). IHC analysis revealed that both SF3B3 and Ki67 immunoreactivities were significantly lower in LoVo-sh SF3B3 #1 xenografts compared to controls (Fig. 2 G). Moreover, LoVo-sh SF3B3 #1 xenografts demonstrated an increase in E-cadherin immunoactivity alongside a reduction in VIM immunoactivity compared to controls (Fig. S 3 D). Secondly, we constructed a CRC lung metastasis models by tail vein injection of LoVo-sh SF3B3 #1 or HT29-sh SF3B3 #2 cells into nude mice (Fig. 2 H). The number and size of metastatic lesions produced by LoVo-sh SF3B3 #1 or HT29-sh SF3B3 #2 cells were significantly decreased compared to their corresponding controls (Fig. 2 I, Fig. S 3 E). Finally, we established a clinically relevant orthotopic mouse model by injecting control and LoVo-sh SF3B3#2 cells into the cecum termini of NSG mice [ 17 ] (Fig. 2 J). Interestingly, SF3B3 knockdown not only suppressed the primary tumor growth in the cecum, but also inhibited the liver metastasis of LoVo cells (Fig. 2 K). Compared to controls, the primary CRC tumor tissues formed in LoVo-sh SF3B3#2 group demonstrated a stronger E-cadherin staining and much weaker VIM staining (Fig. 2 L). Consistently, SF3B3 knockdown led to increased E-cadherin staining and decreased VIM staining in both lung and liver metastasis tumors (Fig. S 3 F). Altogether, these findings suggest that SF3B3 promotes CRC proliferation and metastasis in vivo .
Silencing of SF3B3 induces the intrinsic apoptosis pathway in CRC cells
To elucidate the mechanism underlying SF3B3-mediated regulation of CRC cell growth, we treated SF3B3 -knockdown cells with ZVF (Z-VAD-FMK, a pan-caspase inhibitor of apoptosis), NSA (necrosulfonamide, an inhibitor of necroptosis), or ferroptosis inhibitors (Lip-1, liproxstatin-1; Fer-1, ferrostatin-1). Only the apoptosis inhibitor ZVF significantly restored the growth of SF3B3 -knockdown cells (Fig. S 4 A). Flow cytometric analysis and terminal deoxynucleotidyl transferase-mediated dUTP-fluorescein nick end labeling (TUNEL) staining assays demonstrated a significant promotion of apoptosis in SF3B3 -knockdown LoVo and HT29 cells, which could be rescued by ZVF (Fig. 3 A-B).
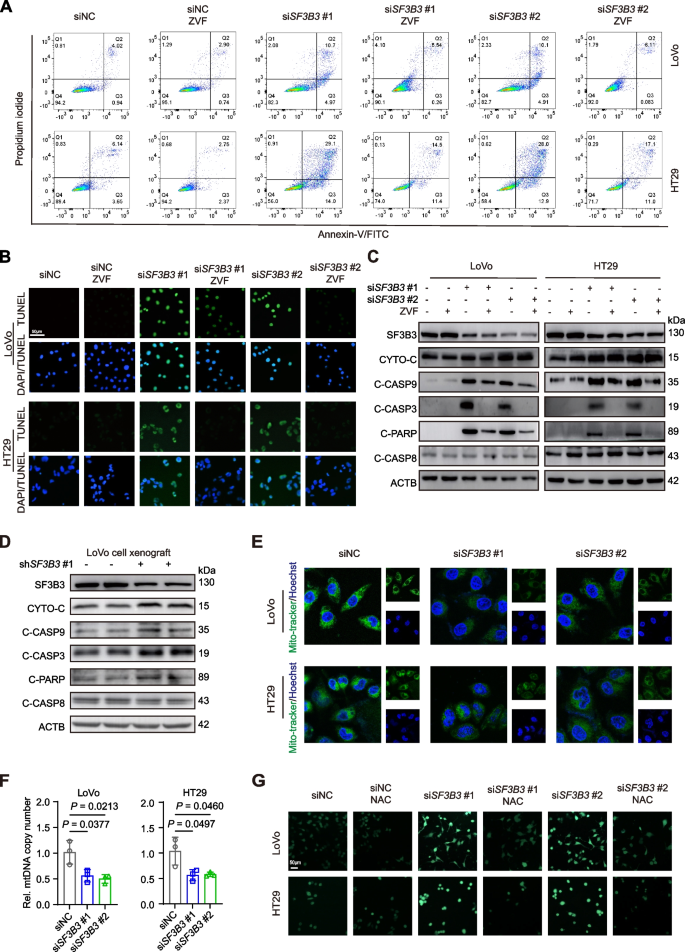
Silencing of SF3B3 induces the intrinsic apoptotic pathway in CRC cells. A Detection of apoptosis by Annexin VFITC/PI staining and flow cytometric analysis. LoVo and HT29 cells were transfected with siRNAs for 48 h followed by 20 μM ZVF (Z-VAD-FMK) treatment for 24 h. B Representative images of TUNEL staining. Nuclei (blue) were stained with DAPI. CRC cells were transfected with siRNAs for 48 h followed by 20 μM ZVF treatment for 24 h. Scale bars, 50 μm. C Representative western blots of apoptosis-related proteins in CRC cells. LoVo and HT29 cells were transfected with siRNAs for 48 h, followed by 20 μM ZVF treatment for 24 h. D Representative western blots of apoptosis-related proteins in LoVo xenografts (shNC vs shSF3B3#1). E Representative fluorescence images of MitoTracker (green) staining using a confocal microscope with 63 × oil immersion lens. Nuclei (blue) were stained with Hoechst 33342. LoVo and HT29 cells were treated with siRNAs for 72 h. F mtDNA copy number was quantified by qRT-PCR. LoVo and HT29 cells were treated with siRNAs for 48 h. Data are shown as mean ± SD. G Representative fluorescence images of ROS (green) staining. LoVo and HT29 cells transfected with siRNAs for 24 h, followed by 10 mM NAC (N-acetylcysteine) treatment for 48 h. Scale bars, 50 μm
There are two major pathways leading to apoptosis. The intrinsic apoptosis pathway is activated by the release of cytochrome C from mitochondria, leading to caspase-9 activation, whereas the extrinsic apoptosis pathway is characterized with caspase-8 activation. Western blot analysis revealed that SF3B3 knockdown significantly increased the protein levels of cytochrome C, cleaved caspase-9, cleaved caspase-3, and cleaved poly ADP-ribose polymerase (PARP), but had no effect on cleaved caspase-8 (Fig. 3 C). Such alterations were markedly rescued by ZVF. Furthermore, the levels of intrinsic apoptosis protein markers were also significantly increased in LoVo-sh SF3B3 xenografts compared to controls (Fig. 3 D).
Mitochondria injury and reactive oxygen species (ROS) are major contributors to the intrinsic apoptosis pathway [ 18 ]. SF3B3 knockdown decreased mitochondrial mass in CRC cells, as revealed by Mito-Tracker staining and mitochondrial DNA (mtDNA) quantification (Fig. 3 E-F). Additionally, ROS production was markedly elevated in SF3B3 -knockdown CRC cells, which was restored by the ROS inhibitor N-acetyl cysteine (NAC) (Fig. 3 G). NAC also markedly rescued the growth and restored the protein levels of apoptosis markers in SF3B3 -knockdown CRC cells (Fig. S 4 B-C). Collectively, these findings suggest that silencing of SF3B3 induces mitochondria-mediated intrinsic apoptosis.
SF3B3 regulates lipogenesis in CRC cells via SREBF1-FASN signaling
To further elucidate the molecular mechanism by which SF3B3 regulated CRC proliferation and metastasis, we conducted RNA-seq of LoVo cells with or without SF3B3 knockdown. A total of 715 significantly upregulated and 419 downregulated genes were identified following SF3B3 knockdown (Table S 3 , Fig. S 5 A). As anticipated, SF3B3 emerged as one of the top significantly downregulated genes by SF3B3 knockdown (Fig. 4 A, Fig. S 5 B). KEGG pathway analysis revealed that the differentially expressed genes due to SF3B3 knockdown were enriched in various pathways, including the “Fatty acid biosynthesis” pathway (Fig. 4 B). SREBF1 (sterol regulatory element binding transcription factor 1, also known as SREBP1) is a master transcriptional regulator for lipogenesis genes, such as ACLY , ACACA , FASN and SCD [ 19 ] (Fig. 4 C). Interestingly, SREBF1 stood out as one of the top 5 most significantly down-regulated genes by SF3B3 knockdown (Fig. 4 A, Table S 3 ). The transcript levels of SREBF1 , ACLY , ACACA , FASN and SCD were all significantly decreased by SF3B3 knockdown (Fig. S 5 C). Such alterations were validated using both qRT-PCR and western blot assays (Fig. 4 D). In contrast, SF3B3 knockdown had no significant effect on SREBF2 , which encodes another SREBP nuclear transcription factor for genes involved cholesterol biosynthesis (Fig. S 5 D). Importantly, the protein levels of SREBF1, ACLY, ACACA, FASN and SCD were also lower in LoVo-sh SF3B3 xenografts compared to controls (Fig. 4 E).
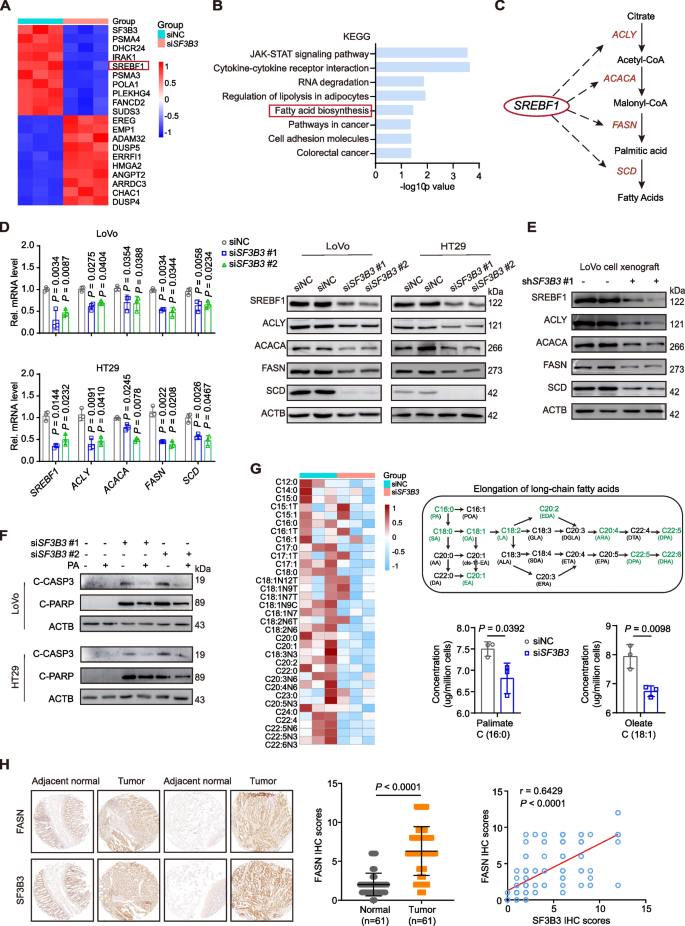
SF3B3 regulates lipogenesis in CRC cells via SREBF1-FASN signaling. A Heatmap displaying the top 10 up- and down-regulated genes from RNA-seq analysis of LoVo cells transiently transfected with siRNAs (si SF3B3 #1 + si SF3B3 #2) for 48 h. B KEGG pathway analysis of SF3B3 -regulated genes from RNA-seq. C Schematic representation of SREBF1-dependent lipogenesis. D mRNA expression of SREBF1 and its target genes, as well as representative western blots of the proteins in SF3B3 -knockdown CRC cells. mRNA levels were detected at 48 h, and protein levels were detected at 72 h after transfection. E Representative western blots of SREBF1, ACLY, ACACA, FASN and SCD in LoVo xenografts (shNC vs sh SF3B3 #1). F Representative western blots of C-CASP3 and C-PARP in SF3B3 -knockdown CRC cells. Cells were transfected with siRNAs for 24 h, then treated with or without 10 μM PA (palmitate) for 48 h. G Lipidomic profiles displaying the fatty acids in LoVo cells transiently transfected with siRNAs (si SF3B3 #1 + si SF3B3 #2) for 72 h. Schematic representation of long-chain fatty acids that were significantly decreased (in green) in SF3B3 -knockdown LoVo cells. H Representative IHC images of FASN in CRC tissues and adjacent normal tissues. The final IHC score was generated by multiplying the score of staining extent with the score of staining intensity. Statistical analysis of FASN IHC scores and correlation analysis with SF3B3 in CRC tissues. Data are shown as mean ± SD
Lipid metabolism reprogramming through SREBF1-FASN axis plays a vital role in the regulation of CRC progression and metastasis [ 20 , 21 , 22 ]. We found that SF3B3 knockdown significantly decreased triglyceride levels in CRC cells (Fig. S 5 E). This was confirmed by Nile red staining (Fig. S 5 F). FASN catalyzes the synthesis of palmitate (PA), which is the starting point for other fatty acids. We found that palmitate could significantly decrease apoptosis biomarkers and ROS in SF3B3 -knockdown CRC cells (Fig. 4 F, Fig. S 5 G). To gain further insights into the role of SF3B3 in CRC metabolism, we utilized mass spectrometry for targeted lipidomic analysis of SF3B3 -knockdown cells. Lipidomic profiles revealed a significant decrease in fatty acids, particularly palmitate (C16:0) and its downstream fatty acids (C18:0, C18:1, C18:2, C20:1, C20:2, C20:4, C22:5, C22:6), in SF3B3 -knockdown cells compared to controls (Fig. 4 G, Fig. S 5 H and Table S 4 ).
TCGA dataset analysis revealed that the mRNA expression of SF3B3 was positively correlated with that of ACLY , ACACA , FASN and SCD in human CRC tissues (Fig. S 5 I). Considering the critical role of FASN in CRC development [ 23 , 24 , 25 ], we assessed FASN protein levels in 61 paired CRC and matched adjacent normal tissues through IHC. We found that FASN protein levels were positively correlated with SF3B3 protein levels in CRC tissues (Fig. 4 H). Altogether, these findings suggest that SF3B3 promotes the malignant phenotype of CRC, at least partially, by regulating SREBF1-FASN-mediated lipogenesis.
SF3B3 regulates SREBF1 via mTOR signaling
SREBF1 comprises two isoforms, namely SREBF1a and SREBF1c, which are transcribed through alternative splicing at transcription start sites, but have distinct regulation of downstream target genes [ 26 ]. The two isoforms differ only in their first exon (exon 1a and exon 1c) (Fig. 5 A). PCR analysis revealed that the decreased SREBF1 mRNA in SF3B3 -knockdown cells was due to the downregulation of SREBF1c mRNA (Fig. 5 B-C). To assess whether SF3B3 is involved in the promoter splicing of SREBF1 , we performed RNA immunoprecipitation (RIP) assays, using the known SF3B3 target gene EZH2 as the positive control [ 27 ]. Notably, we did not detect SREBF1 mRNA in the SF3B3 protein-antibody-bead system (Fig. 5 D). This suggests that SF3B3-mediated regulation of SREBF1c expression does not stem from the alternative splicing of SREBF1 promoter.
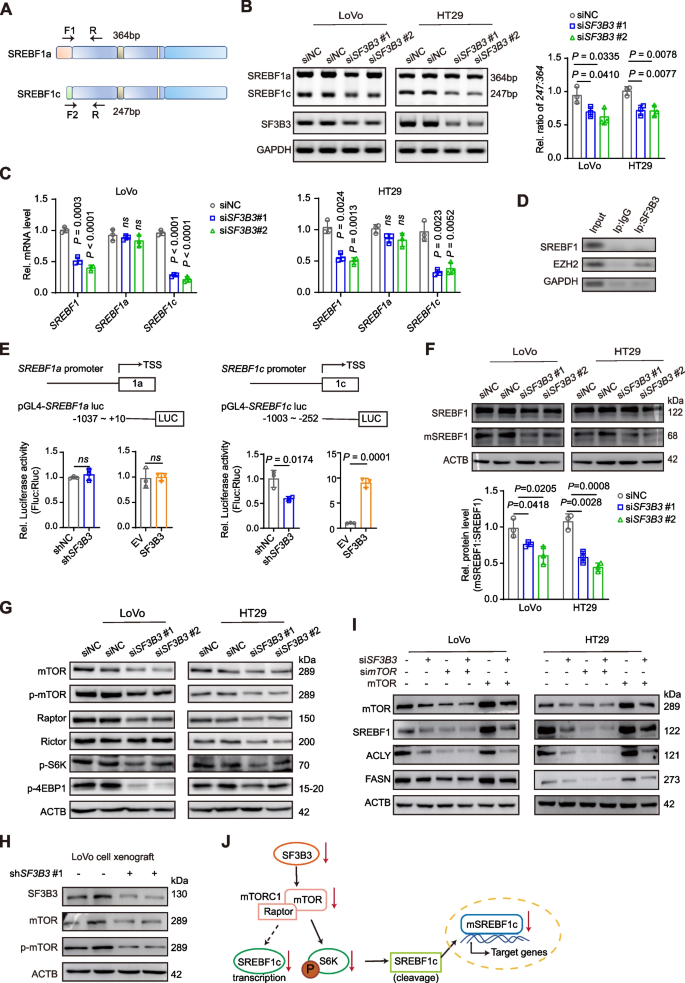
SF3B3 regulates SREBF1 via mTOR signaling. A Schematic representation of PCR primers used for the identification of SREBF1a and SREBF1c . B Relative SREBF1a and SREBF1c mRNA levels were examined and analyzed by 3% agarose gel electrophoresis of PCR products. LoVo and HT29 cells were treated with siRNAs for 48 h. C The mRNA expression of SREBF1a and SREBF1c was determined by qRT-PCR. D The immunoprecipitated RNAs by anti-SF3B3 and negative IgG were purified and analyzed by PCR using primers specific for SREBF1 and EZH2 . E Schematic diagram illustrating the SREBF1a and SREBF1c promoters. Dual luciferase reporter assay was used to determine SREBF1a and SREBF1c promoter activity in HEK293T cells after infection with sh SF3B3 #1 lentivirus (shNC vs sh SF3B3 ) or transfection with overexpressing plasmids (empty vector, EV; SF3B3 overexpressing plasmid, SF3B3). F Western blot analysis of full-length SREBF1 and mature SREBF1 (mSREBF1) in SF3B3 -knockdown CRC cells. LoVo and HT29 cells were treated with siRNAs for 72 h. G Representative western blots of mTOR, p-mTOR, Raptor, Rictor, p-S6K and p-4EBP1 in SF3B3 -knockdown CRC cells. LoVo and HT29 cells were treated with siRNAs for 72 h. H Representative western blots of mTOR and p-mTOR in LoVo xenografts (shNC vs sh SF3B3 #1). I Representative western blots of mTOR, SREBF1, ACLY, and FASN in SF3B3 -knockdown CRC cells after mTOR silencing or overexpression. LoVo and HT29 cells were treated with siRNAs for 12 h, followed by transfection with either si mTOR or mTOR -overexpressing plasmids for 60 h. J Schematic diagram illustrating the role of mTOR in regulating SREBF1c in SF3B3 -knockdown CRC cells. Data are shown as mean ± SD
Next, we determined whether SF3B3 regulated SREBF1 at the transcription or protein level. The promoter DNA fragments (~ 1000 bp upstream of the first exon) of SREBF1a and SREBF1c were constructed into dual luciferase report vectors. SREBF1a promoter activity was not altered by SF3B3 knockdown or over-expression. In contrast, SREBF1c promoter activity was positively associated with SF3B3 expression (Fig. 5 E). At the protein level, SREBF1 activation involves the proteolytic cleavage of the N-terminal half of the protein followed by nuclear translocation. Intriguingly, SF3B3 knockdown significantly decreased the ratio of mature SREBF1 to full-length SREBF1 in CRC cells (Fig. 5 F). These findings suggest that SF3B3 regulate SREBF1c in CRC cells through both gene transcription and protein cleavage.
Because mTOR has been reported to regulate both SREBF1c transcription and activation [ 28 , 29 , 30 ], we determined whether mTOR was involved in SF3B3-mediated regulation of SREBF1c. Notably, SF3B3 knockdown significantly decreased the protein levels of both p-mTOR and mTOR in CRC cells (Fig. 5 G). Furthermore, LoVo-sh SF3B3 xenografts also showed lower p-mTOR and mTOR levels compared to controls (Fig. 5 H). mTOR is a critical component of two multiprotein signaling complexes named mTORC1 and mTORC2. Functionally, mTORC1 is considered a master regulator of cell growth and proliferation, whereas mTORC2 primarily regulates cytoskeletal structure and cell survival [ 31 ]. We found that SF3B3 knockdown decreased the protein level of Raptor, a component of mTORC1, but had no significant effect on the protein level of Rictor, a component of mTORC2. Consistently, SF3B3 knockdown decreased the protein levels of two mTORC1 downstream effectors, pS6K and 4EBP1 (Fig. 5 G). Furthermore, we found that the downregulation of SREBF1 and its target genes ( ACLY and FASN ) by SF3B3 knockdown was not prominent in mTOR -knockdown cells, and could be largely restored by mTOR overexpression (Fig. 5 I). The tuberous sclerosis protein complex (TSC complex) is a critical negative regulator of mTOR [ 32 ]. We found that knockdown of both TSC1 and TSC2 could significantly increase the mRNA and protein expression of SREBF1 , ACLY and FASN in SF3B3 -knockdown CRC cells (Fig. S 5 J-K). Collectively, these findings suggest that mTORC1 regulates the gene transcription and protein cleavage of SREBF1c in SF3B3 -knockdown CRC cells (Fig. 5 J).
SF3B3 regulates mTOR splicing
Because SF3B3 knockdown significantly suppressed the mRNA expression of mTOR in CRC cells (Fig. S 6 A-B), we determined whether SF3B3 was involved in mTOR RNA splicing. Firstly, we assessed the impact of SF3B3 knockdown on AS events in CRC cells by analyzing RNA-seq data. A total of 20,736 AS events were significantly altered by SF3B3 knockdown, encompassing alternative 3’ splice site (ss) exon (A3SS), alternative 5’ ss exon (A5SS), mutually exclusive exons (MXE), retained intron (RI), and skipped exon (SE) events (Fig. 6 A). Notably, more than 60% of these AS events corresponded to skipped exon (SE). KEGG pathway analysis indicated that SF3B3 -regualted AS events were most significantly enriched in genes involved in “Metabolic pathways” and “Spliceosome”, which was consistent with the mRNA analysis using TCGA datasets (Fig. S 6 C). Secondly, we investigated whether SF3B3 knockdown altered mTOR isoforms. To date, only two functional human mTOR isoforms, namely mTORα and mTORβ, have been identified [ 10 , 33 ]. We used the same PCR primers and antibodies reported in previous study for detecting mTORβ (Fig. 6 B, Fig. S 6 D). Strikingly, SF3B3 knockdown increased both mRNA and protein levels of mTORβ in CRC cells (Fig. 6 B-C). Thirdly, we analyzed the sequences of three protein-coding mTOR transcript variants ( mTOR variant 1, NM_004958.4; mTOR variant 2, NM_001386500.1; mTOR variant 3, NM_004958.4) in NCBI database. mTOR variant 1 and variant 2 encode the same isoform, but differ from mTOR variant 3 at exon 8, where skipping occurs (Fig. 6 D). Notably, exon 8 is also skipped in mTORβ transcript. We compared the rescue effects of full-length mTOR and mTOR variant 3 in SF3B3 -knockdown CRC cells. Both cell viability and SREBF1 protein levels of SF3B3 -knockdown cells could be significantly increased upon overexpression of full-length mTOR (+ exon 8), whereas overexpression of mTOR variant 3 (-exon 8) did not elicit a similar rescue effect (Fig. S 6 E). This suggests that exon 8-skipped splicing may attenuate the rescuing capability of mTOR in SF3B3 -knockdown CRC cells.
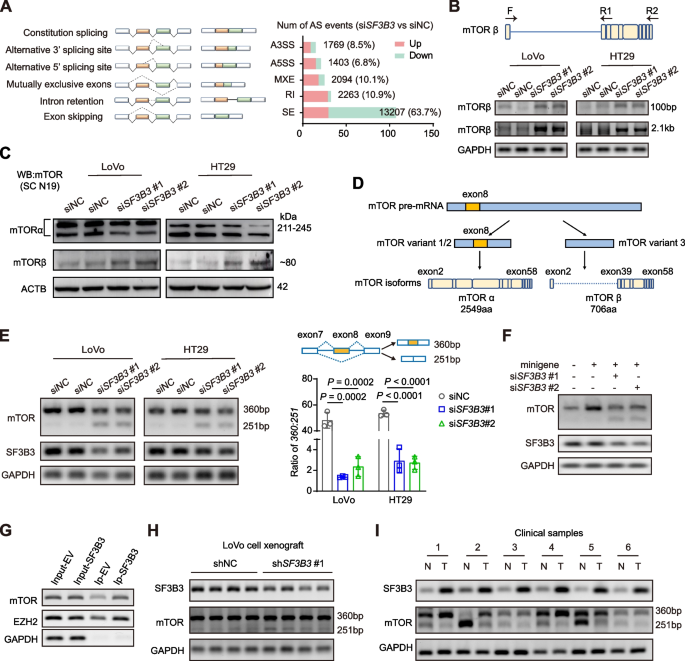
SF3B3 regulates mTOR exon 8 skipping in CRC cells. A Schematic diagram of alternative splicing types, and alternative splicing events regulated by SF3B3 from RNA-seq data. B The mTORβ transcript levels were examined by 1% agarose gel electrophoresis of PCR products. The 2.1 kb product (full-length mTORβ transcript) was amplified with the primers (F, R2), whereas the 100 bp product (partial mTORβ transcript) was amplified with the primers (F, R1). C Representative western blots of full-length mTOR (mTORα) and its short-length isoform (mTORβ) in CRC cells using the mTOR antibody (sc-517464). LoVo and HT29 cells were treated with siRNAs for 72 h. D Schematic diagram of the protein-coding mTOR transcript variants and isoforms. mTOR variant 1 and variant 2 include exon 8, whereas mTOR variant 3 exclude exon 8 in NCBI dataset. Exon 8 is also skipped in mTORβ transcript. E Exon 8 skipping of mTOR was examined by 3% agarose gel electrophoresis of PCR products in CRC cells. LoVo and HT29 cells were treated with siRNAs for 48 h. Data are shown as mean ± SD. F Exon 8 skipping was determined by 3% agarose gel electrophoresis of PCR products in CRC cells after 48 h transfection with a minigene contains exons 7–9 as well as two introns of mTOR . G The immunoprecipitated RNAs by anti-SF3B3 or IgG were purified and analyzed by PCR using primers specific for mTOR and EZH2 . EZH2 used as positive control. H 3% agarose gel electrophoresis of PCR products of mTOR exon 8 skipping and SF3B3 mRNA in control and SF3B3 -knockdown LoVo xenografts (shNC vs sh SF3B3 #1). I Representative agarose gel electrophoresis of PCR products of mTOR exon 8 skipping and SF3B3 mRNA in paired CRC tissues and adjacent normal tissues
We then investigated whether SF3B3 regulated exon 8 skipped splicing of mTOR . PCR analysis revealed that SF3B3 knockdown markedly increased endogenous mTOR exon 8 skipping in CRC cells (Fig. 6 E). The effect of SF3B3 knockdown on exon 8 skipping was further validated in LoVo cells transfected with a minigene recombinant plasmid containing exons 7–9 of mTOR genomic DNA (Fig. 6 F). We also examined the impact of SF3B3 overexpression on mTOR splicing. As expected, SF3B3 overexpression notably decreased mTOR exon 8 skipping in SW480 cells, with a concomitant decrease in mTORβ protein level (Fig. S 6 F). RIP analysis revealed that mTOR mRNA could be immunoprecipitated with the SF3B3 protein-antibody-bead system, which was higher in SF3B3 overexpressing cells than in controls (Fig. 6 G, Fig. S 6 G). Finally, we assessed the role of SF3B3 in regulating mTOR splicing in vivo. SF3B3 knockdown increased exon 8 skipping of mTOR in LoVo cell xenografts (Fig. 6 H). A higher mTOR exon 8 skipping was observed in tumor tissues compared to matched normal tissues, showing a negative correlation with SF3B3 expression (Fig. 6 I). Collectively, these findings suggest that SF3B3 regulates exon 8 skipped splicing of mTOR RNA.
SF3B3 regulates autophagy
Because mTOR is a master regulator of autophagy, we determined the impact of SF3B3 on autophagy. SF3B3 knockdown in CRC cells significantly increased the accumulation of LC3B, a specific marker for autophagosomes, as revealed by both western blot and immunofluorescence analyses (Fig. 7 A, Fig. S 6 H). The induction of LC3B in SF3B3 -knockdown CRC cells could be restored by mTOR overexpression (Fig. S 6 I). SF3B3 knockdown also significantly decreased the protein level of SQSTM1/p62, a marker for autophagic flux (Fig. 7 A). By using the Lenti-mCherry-GFP-LC3B fluorescent assay, we found that SF3B3 knockdown increased both autophagosomes and autophagolysosomes in CRC cells (Fig. 7 B). Cloroquine (CQ) and Bafilomycin A1 (BafA1), two autophagy inhibitors that prevent the fusion of autophagosome with lysosome, significantly increased the accumulation of LC3B in SF3B3 -knockdown CRC cells (Fig. S 6 J). By using transmission electron microscopy, we observed the autophagic vacuoles (double-membrane compartments containing lamellar structures) in SF3B3 -knockdown CRC cells (Fig. 7 C). These findings suggest that SF3B3 knockdown induces autophagic flux in CRC cells.
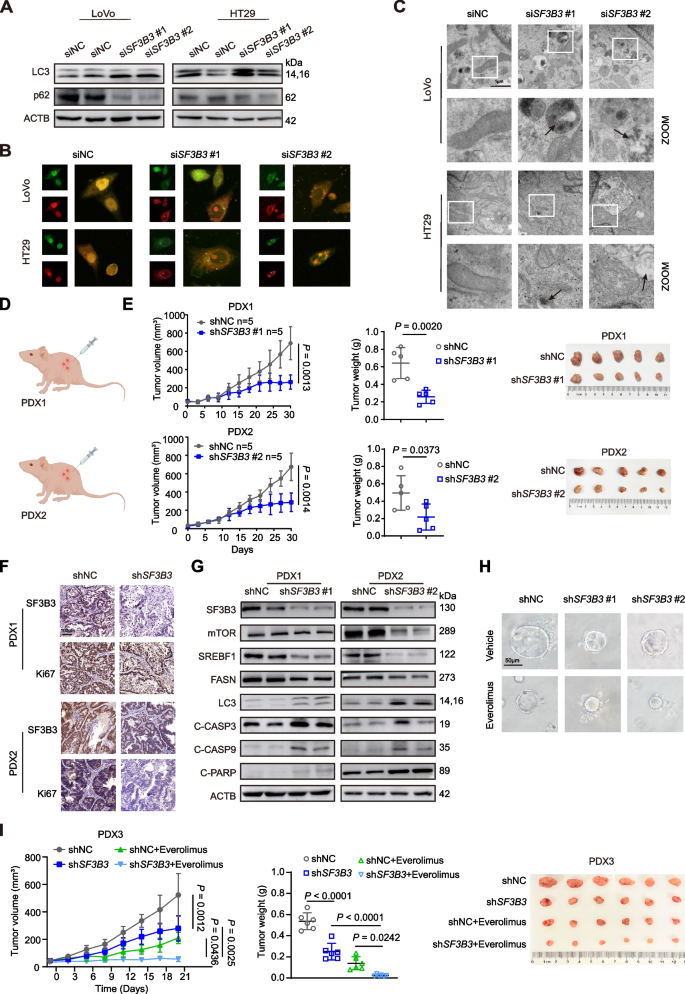
SF3B3 knockdown and mTOR inhibitor induce synergistic antitumor activity. A Representative western blots of LC3 and SQSTM1/p62 in SF3B3 -knockdown CRC cells. LoVo and HT29 cells were treated with siRNAs for 72 h. B Representative autophagic flux images. mCherry-GFP-LC3B labeled CRC cells were transfected with SF3B3 siRNAs for 48 h. C Representative transmission electron microscopy images presenting the ultrastructure of the SF3B3 -knockdown CRC cells. Arrows indicate autophagic vacuoles. LoVo and HT29 cells were treated with siRNAs (si SF3B3 #1 + si SF3B3 #2) for 72 h. Scale bars, 1 µm. D Schematic diagram of two PDX models. PDX1 models were treated Lentivirus-shNC vs Lentivirus-sh SF3B3 #1, and PDX2 models were treated Lentivirus-shNC vs Lentivirus-sh SF3B3 #2. E Tumor growth, tumor weights, and tumor photos of two PDX mouse models after intratumor injection of shNC or sh SF3B3 lentivirus. F Representative IHC images of SF3B3 and Ki67 in two PDX tumor tissues. G Representative western blots of lipogenesis-, autophagy- and apoptosis-related proteins in PDX tumor tissues. H Representative images of patient-derived CRC tumor organoids after treatment with SF3B3 shRNA lentivirus and everolimus. Digested organoids were transduced with sh SF3B3 lentivirus for 6 h, followed by reconstitution in matrigel in 24-well plate. After culturing for 7 days, organoids were further treated with or without 40 μM everolimus for 48 h. Scale bars, 50 μm. I Tumor growth, tumor weights, and photos of PDX3 tumors. Mice were intratumorally injected with shNC or sh SF3B3 lentivirus (sh SF3B3 #1 + sh SF3B3 #2), followed by oral gavage of vehicle (40%PEG400, 5% Tween-80 and 5% DMSO) or everolimus (5 mg/kg, p.o., every two days for 3 weeks). Data are shown as mean ± SD
Pan-cancer analysis of TCGA data revealed that SF3B3 expression correlated positively with the expression of full-length mTOR -001 isoform (Fig. S 6 K). We then silenced SF3B3 in breast cancer cells (MCF7), cervical cancer cells (Hela) and liver cancer cells (Huh7). Notably, SF3B3 knockdown markedly increased exon 8 skipping of mTOR and LC3B protein levels in all three cancer cell lines (Fig. S 6 L-M). These findings suggest that SF3B3 plays a critical role in regulating mTOR splicing and autophagy in multiple cancers.
SF3B3 knockdown and mTOR inhibitor induce synergistic antitumor activity
To assess the therapeutic potential of targeting SF3B3 in CRC, we constructed two CRC patient-derived xenograft (PDX) models. The clinical information of donor patients was provided in Fig. S 7 A. The two PDX models were administered sh SF3B3 #1 and sh SF3B3 #2 lentiviruses, respectively, via intratumor injection (Fig. 7 D). SF3B3 knockdown in two PDX murine models was validated by qRT-PCR (Fig. S 7 B). Compared to shNC control groups, the volume and growth rate of tumors in sh SF3B3 groups were significantly decreased (Fig. 7 E). IHC staining showed that Ki67 levels markedly decreased in sh SF3B3 groups (Fig. 7 F). A significant increase of apoptotic cells was observed in sh SF3B3 groups using TUNEL assay (Fig. S 7 C). Western blot analysis revealed that SF3B3 inhibition markedly reduced protein levels of mTOR, SREBF1 and FASN (Fig. 7 G). Additionally, the autophagy- and apoptosis-related markers were significantly elevated in sh SF3B3 groups compared to shNC groups.
Notably, silencing of SF3B3 significantly increased the sensitivity of two mTOR inhibitors, namely rapamycin and everolimus, in LoVo and HT29 cells (Fig. S 7 D). We then determined whether SF3B3 inhibition could be combined with mTOR inhibitors for CRC treatment in patient-derived CRC organoids (Fig. S 7 E). Interestingly, the combination of sh SF3B3 lentivirus and everolimus markedly inhibited the CRC organoid growth (Fig. 7 H). Furthermore, a third PDX mouse model was constructed, and mice were treated with sh SF3B3 lentivirus by intratumor injection, followed by oral administration of everolimus. Notably, the combination treatment induced synergistically inhibitory effects on PDX growth, without affecting the body weights (Fig. 7 I, Fig. S 7 F-G). Altogether, these findings suggest that SF3B3 is a potential therapeutic target for CRC treatment (Fig. 8 ).
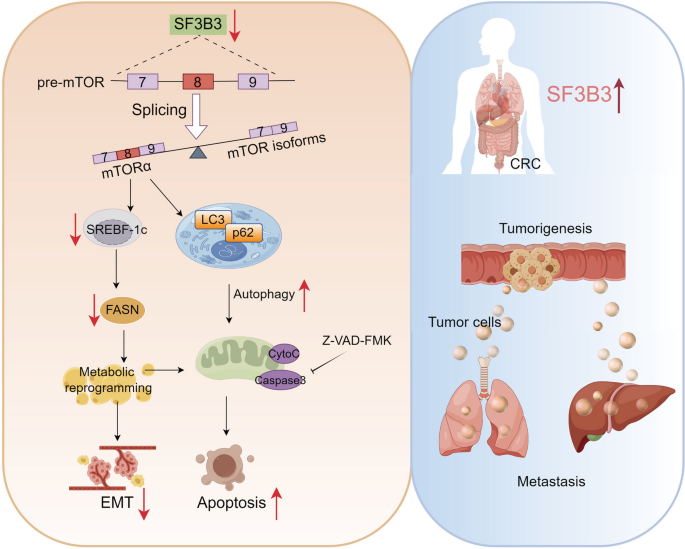
Mechanistic model illustrating the role of SF3B3 in CRC. SF3B3 is upregulated in CRC tissues, promoting CRC tumorigenesis and metastasis. Targeting SF3B3 through RNA silencing downregulates mTORα via alternative splicing, resulting in the induction of autophagy, suppression of SREBF1 transcription, and inhibition of SREBF1 protein activation. Consequently, silencing of SF3B3 prevents CRC progression and metastasis by inhibiting lipogenesis and promoting apoptosis
The SF3b complex plays key roles in spliceosome assembly and activation. Among its seven components, SF3B1 is considered the most commonly mutated splicing factor across cancers [ 34 ]. The functions of SF3B1 and its inhibitors have been extensively evaluated in various cancers. In contrast, SF3B3 mutations are rare in cancers, and the functional role of SF3B3 in cancer development remains largely unknown. SF3B3 expression is upregulated in renal cancer, and is associated with tumor stage and poor prognosis[ 35 ]. Elevated expression of SF3B3 correlates with poor prognosis and tamoxifen resistance in ER-positive breast cancer [ 36 ]. Interestingly, knockdown of SF3B3 inhibits the cell growth of both renal cancer cells and breast cancer cells. In this study, we demonstrate that SF3B3 is overexpressed in CRC, representing a potential therapeutic target for CRC treatment.
One of the major findings in this study is the critical role of SF3B3 in regulating alternative splicing of mTOR mRNA. Mechanistic studies on mTOR alternative splicing have been limited thus far. Src associated in mitosis (SAM68) was reported to promote splicing at the 5’ splice site in intron 5 of mTOR by interacting with U1 small nuclear ribonucleoprotein (U1 snRNP) [ 37 , 38 ]. It should be noted that this short-length premature mTOR isoform is not functional. Various mTOR transcripts have been listed in different databases. A total of 7 mTOR isoforms are identified in TCGA datasets (mTOR-001 to mTOR-007) (Fig. S 6 I). The full-length mTOR -001 and the short-length mTOR -002 are highly expressed in tumors. Similar as functional mTORβ isoform, exon 8 is skipped in mTOR-002. In this study, we identify SF3B3 as a key mechanism for the formation of mTORβ and other exon 8-skipped mTOR isoforms. Additional research is necessary to explore the involvement of various mTOR isoforms in SF3B3-mediated progression and metastasis of CRC cells.
The findings in this study are significant for the development of mTOR inhibitors, which have been developed and evaluated in clinical trials for various cancer types. First, skipping exons in nucleotide sequence may lead to disruption of the drug-binding site, which may affect the efficacy and resistance of drugs. For instance, mTORβ -overexpressing cells is less sensitive to the inhibitory effect of rapamycin compared to mTORα-overexpressing cells [ 10 ]. Therefore, a better understanding of mTOR splicing may enhance the success of mTOR inhibitor development. Second, our results suggest a critical role of SF3B3 in regulating mTOR expression. A positive correlation between SF3B3 and the full length mTOR isoform ( mTOR -001) was identified in multiple cancers based on analysis of TCGA datasets. Therefore, mTOR inhibitors might be helpful in the treatment of SF3B3 highly expressed cancers. Third, inhibiting multiple nodes of a pathway, either downstream or upstream of the driver oncogene, has proven a rational and promising approach for tumor therapy. Our findings suggest that combination treatment of both SF3B3 and mTOR inhibitors may represent a promising approach for CRC therapy.
This study identifies SF3B3 as a negative autophagic regulator across various cancers. Interestingly, knockdown of SF3B3 was also shown to increase the expression levels of LC3B in neural cells [ 39 ]. This may suggest that SF3B3-regualted autophagy is not limited to tumor cells. A previous study reported that SF3B3 knockdown induces autophagy in breast cancer cells, but the underlying mechanism is not fully elucidated [ 40 ]. This study demonstrates that SF3B3-regualted mTOR splicing contributes to autophagy in SF3B3 -knockdown breast cancer cells. Autophagy plays context-dependent roles in various types of cancers [ 41 ]. In breast cancer cells, autophagy induced by SF3B3 knockdown inhibits the proliferation and migration of breast cancer cells without inducing apoptosis [ 40 ]. Consistently, autophagy inhibitors show little effect on cell death (apoptosis) of SF3B3 -knockdown CRC cells (data not shown). Future studies are needed to elucidate the exact role of autophagy in SF3B3 -knockdown CRC cells.
Lipid metabolism reprogramming is a hallmark of cancer cells. In normal epithelial cells, fatty acids can be obtained from diet or synthesized de novo from carbohydrate precursors. In contrast, most fatty acids in tumor cells are derived from de novo lipogenesis [ 42 ]. SREBF1 and FASN are two central regulators of lipogenesis. SREBF1 mainly regulates the transcription of lipogenic genes. FASN is the key rate-limiting enzyme in de novo lipogenesis and catalyzes the formation of palmitate, the first fatty acid product in de novo lipogenesis. Both SREBF1 and FASN are significantly upregulated in CRC tissues compared with normal tissues [ 21 , 43 ]. Inhibition of SREBF1 or FASN has been shown to inhibit CRC cell growth [ 20 , 44 ]. Interestingly, SREBF1 is associated with the invasion and metastasis of CRC cells [ 21 ], whereas FASN plays a key role in regulating cell respiration [ 44 ]. This study suggests a critical role of SF3B3 in regulating lipid metabolism in CRC cells via SREBF1-FASN axis.
As SF3B3 knockdown leads to alterations in numerous splicing events, it is unlikely that a single splicing event fully explains the role of SF3B3 in CRC. For instance, the increased ROS in SF3B3 -knockdown CRC cells could be a consequence of multiple pathways, such as SF3B3-DHCR24 axis. Our RNA-seq data showed that DHCR24 was among the top 5 most significantly down-regulated genes by SF3B3 knockdown in CRC cells (Table S 3 ). The downregulation of DHCR24 expression in CRC cells by SF3B3 knockdown was validated using qRT-PCR and western blot (Fig. S 8 A). Further study showed that SF3B3 knockdown promoted the retention of intron between exon 3 and 4 of DHCR24 , indicating a critical role of SF3B3 in regulating DHCR24 splicing (Fig. S 8 B). DHCR24 is reported to function as a hydrogen peroxide scavenger and plays an oncogenic role in various cancers [ 45 , 46 ]. We found that reinforced expression of DHCR24 significantly promoted cell growth and decreased the ROS production level of SF3B3 -knockdown CRC cells (Fig. S 8 C-D). Furthermore, splicing factors such as SR and hnRNP proteins have been reported to regulate all levels of apoptotic gene expression, including transcription, alternative splicing, mRNA stability, translation, and protein stability [ 47 ]. We evaluated the effect of SF3B3 knockdown on several critical apoptotic genes that have been previously documented to undergo alternative splicing events [ 48 ]. Notably, SF3B3 knockdown increased the formation of pro-apoptotic mRNA isoform of MCL1 (encoding myeloid cell leukemia-1) and CASP9 (encoding caspase-9), both crucial genes involved in the intrinsic pathway of apoptosis (Fig. S 8 E). Therefore, it is likely numerous SF3B3 targets contribute to its phenotypic effects (such as apoptosis), and depletion of SF3B3 could impact on multiple different pathways.
Our in vitro and in vivo investigations demonstrated the importance of SF3B3 in promoting CRC progression and metastasis through regulating mTOR splicing and lipid metabolism (Fig. 8 ). Targeting SF3B3 by RNA silencing showed synergistic antitumor effects when combined with mTOR inhibitors. Additional studies in other cancer types may be investigated to test whether targeting SF3B3 could be broadly applied.
Availability of data and materials
The data that support the findings of this study are available upon request.
Abbreviations
- Colorectal cancer
Chromatin immunoprecipitation sequencing
Epithelial-mesenchymal transition
Empty vector
Mitochondrial DNA
Mammalian or mechanistic target of rapamycin
N-acetyl cysteine
Transmission electron microscopy
Patient-derived xenograft
RNA immunoprecipitation
Reactive oxygen species
Sterol regulatory element binding transcription factor 1
Siegel RL, Wagle NS, Cercek A, Smith RA, Jemal A. Colorectal cancer statistics, 2023. CA Cancer J Clin. 2023;73:233–54.
Article PubMed Google Scholar
Zarour LR, Anand S, Billingsley KG, Bisson WH, Cercek A, Clarke MF, et al. Colorectal Cancer Liver Metastasis: Evolving Paradigms and Future Directions. Cell Mol Gastroenterol Hepatol. 2017;3:163–73.
Article PubMed PubMed Central Google Scholar
Scotti MM, Swanson MS. RNA mis-splicing in disease. Nat Rev Genet. 2016;17:19–32.
Article CAS PubMed Google Scholar
Chen Y, Huang M, Liu X, Huang Y, Liu C, Zhu J, et al. Alternative splicing of mRNA in colorectal cancer: new strategies for tumor diagnosis and treatment. Cell Death Dis. 2021;12:752.
Xu T, Verhagen M, Joosten R, Sun W, Sacchetti A, Munoz Sagredo L, et al. Alternative splicing downstream of EMT enhances phenotypic plasticity and malignant behavior in colon cancer. Elife. 2022;11:e82006.
Article CAS PubMed PubMed Central Google Scholar
Sun Q, Han Y, He J, Wang J, Ma X, Ning Q, et al. Long-read sequencing reveals the landscape of aberrant alternative splicing and novel therapeutic target in colorectal cancer. Genome Med. 2023;15:76.
Tian T, Li X, Zhang J. mTOR signaling in cancer and mTOR inhibitors in solid tumor targeting therapy. Int J Mol Sci. 2019;20:755.
Faller WJ, Jackson TJ, Knight JR, Ridgway RA, Jamieson T, Karim SA, et al. mTORC1-mediated translational elongation limits intestinal tumour initiation and growth. Nature. 2015;517:497–500.
Wang H, Liu Y, Ding J, Huang Y, Liu J, Liu N, et al. Targeting mTOR suppressed colon cancer growth through 4EBP1/eIF4E/PUMA pathway. Cancer Gene Ther. 2020;27:448–60.
Panasyuk G, Nemazanyy I, Zhyvoloup A, Filonenko V, Davies D, Robson M, et al. mTORbeta splicing isoform promotes cell proliferation and tumorigenesis. J Biol Chem. 2009;284:30807–14.
Sun C. The SF3b complex: splicing and beyond. Cell Mol Life Sci. 2020;77:3583–95.
Ding N, Li M, Zhao X. PHF5A is a potential diagnostic, prognostic, and immunological biomarker in pan-cancer. Sci Rep. 2023;13:17521.
Komor MA, Pham TV, Hiemstra AC, Piersma SR, Bolijn AS, Schelfhorst T, et al. Identification of Differentially Expressed Splice Variants by the Proteogenomic Pipeline Splicify. Mol Cell Proteomics. 2017;16:1850–63.
Chang Y, Zhao Y, Wang L, Wu M, He C, Huang M, et al. PHF5A promotes colorectal cancerprogression by alternative splicing of TEAD2. Mol Ther Nucleic Acids. 2021;26:1215–27.
Wang Z, Yang X, Liu C, Li X, Zhang B, Wang B, et al. Acetylation of PHF5A Modulates Stress Responses and Colorectal Carcinogenesis through Alternative Splicing-Mediated Upregulation of KDM3A. Mol Cell. 2019;74:1250–63.
Mei S, Qin Q, Wu Q, Sun H, Zheng R, Zang C, et al. Cistrome Data Browser: a data portal for ChIP-Seq and chromatin accessibility data in human and mouse. Nucleic Acids Res. 2017;45:D658–62.
Zhang L, Zhu Z, Yan H, Wang W, Wu Z, Zhang F, et al. Creatine promotes cancer metastasis through activation of Smad2/3. Cell Metab. 2021;33:1111–23.
Redza-Dutordoir M, Averill-Bates DA. Activation of apoptosis signalling pathways by reactive oxygen species. Biochim Biophys Acta. 2016;1863:2977–92.
Shimano H, Sato R. SREBP-regulated lipid metabolism: convergent physiology - divergent pathophysiology. Nat Rev Endocrinol. 2017;13:710–30.
Wen YA, Xiong X, Zaytseva YY, Napier DL, Vallee E, Li AT, et al. Downregulation of SREBP inhibits tumor growth and initiation by altering cellular metabolism in colon cancer. Cell Death Dis. 2018;9:265.
Gao Y, Nan X, Shi X, Mu X, Liu B, Zhu H, et al. SREBP1 promotes the invasion of colorectal cancer accompanied upregulation of MMP7 expression and NF-kappaB pathway activation. BMC Cancer. 2019;19:685.
Zaytseva YY, Rychahou PG, Gulhati P, Elliott VA, Mustain WC, O’Connor K, et al. Inhibition of fatty acid synthase attenuates CD44-associated signaling and reduces metastasis in colorectal cancer. Cancer Res. 2012;72:1504–17.
Lu T, Sun L, Wang Z, Zhang Y, He Z, Xu C. Fatty acid synthase enhances colorectal cancer cell proliferation and metastasis via regulating AMPK/mTOR pathway. Onco Targets Ther. 2019;12:3339–47.
Wang H, Xi Q, Wu G. Fatty acid synthase regulates invasion and metastasis of colorectal cancer via Wnt signaling pathway. Cancer Med. 2016;5:1599–606.
Wei W, Qin B, Wen W, Zhang B, Luo H, Wang Y, et al. FBXW7beta loss-of-function enhances FASN-mediated lipogenesis and promotes colorectal cancer growth. Signal Transduct Target Ther. 2023;8:187.
Harada N, Yonemoto H, Yoshida M, Yamamoto H, Yin Y, Miyamoto A, et al. Alternative splicing produces a constitutively active form of human SREBP-1. Biochem Biophys Res Commun. 2008;368:820–6.
Chen K, Xiao H, Zeng J, Yu G, Zhou H, Huang C, et al. Correction: Alternative Splicing of EZH2 pre-mRNA by SF3B3 Contributes to the Tumorigenic Potential of Renal Cancer. Clin Cancer Res. 2022;28:1736.
Duvel K, Yecies JL, Menon S, Raman P, Lipovsky AI, Souza AL, et al. Activation of a metabolic gene regulatory network downstream of mTOR complex 1. Mol Cell. 2010;39:171–83.
Porstmann T, Santos CR, Griffiths B, Cully M, Wu M, Leevers S, et al. SREBP activity is regulated by mTORC1 and contributes to Akt-dependent cell growth. Cell Metab. 2008;8:224–36.
Yi J, Zhu J, Wu J, Thompson CB, Jiang X. Oncogenic activation of PI3K-AKT-mTOR signaling suppresses ferroptosis via SREBP-mediated lipogenesis. Proc Natl Acad Sci U S A. 2020;117:31189–97.
Saxton RA, Sabatini DM. mTOR Signaling in Growth, Metabolism, and Disease. Cell. 2017;169:361–71.
Garami A, Zwartkruis FJ, Nobukuni T, Joaquin M, Roccio M, Stocker H, et al. Insulin activation of Rheb, a mediator of mTOR/S6K/4E-BP signaling, is inhibited by TSC1 and 2. Mol Cell. 2003;11:1457–66.
Ibrahim YH, Pantelios S, Mutvei AP. An affinity tool for the isolation of endogenous active mTORC1 from various cellular sources. J Biol Chem. 2023;299:104644.
Yamauchi H, Nishimura K, Yoshimi A. Aberrant RNA splicing and therapeutic opportunities in cancers. Cancer Sci. 2022;113:373–81.
Chen K, Xiao H, Zeng J, Yu G, Zhou H, Huang C, et al. Alternative Splicing of EZH2 pre-mRNA by SF3B3 Contributes to the Tumorigenic Potential of Renal Cancer. Clin Cancer Res. 2017;23:3428–41.
Gokmen-Polar Y, Neelamraju Y, Goswami CP, Gu X, Nallamothu G, Janga SC, et al. Expression levels of SF3B3 correlate with prognosis and endocrine resistance in estrogen receptor-positive breast cancer. Mod Pathol. 2015;28:677–85.
Subramania S, Gagne LM, Campagne S, Fort V, O’Sullivan J, Mocaer K, et al. SAM68 interaction with U1A modulates U1 snRNP recruitment and regulates mTor pre-mRNA splicing. Nucleic Acids Res. 2019;47:4181–97.
Huot ME, Vogel G, Zabarauskas A, Ngo CT, Coulombe-Huntington J, Majewski J, et al. The Sam68 STAR RNA-binding protein regulates mTOR alternative splicing during adipogenesis. Mol Cell. 2012;46:187–99.
Wang J, Weng Y, Li Y, Zhang Y, Zhou J, Tang J, et al. The interplay between lncRNA NR_030777 and SF3B3 in neuronal damage caused by paraquat. Ecotoxicol Environ Saf. 2023;255:114804.
Zhang S, Zhang J, An Y, Zeng X, Qin Z, Zhao Y, et al. Multi-omics approaches identify SF3B3 and SIRT3 as candidate autophagic regulators and druggable targets in invasive breast carcinoma. Acta Pharm Sin B. 2021;11:1227–45.
Rybstein MD, Bravo-San Pedro JM, Kroemer G, Galluzzi L. The autophagic network and cancer. Nat Cell Biol. 2018;20:243–51.
Menendez JA, Lupu R. Fatty acid synthase and the lipogenic phenotype in cancer pathogenesis. Nat Rev Cancer. 2007;7:763–77.
Ogino S, Nosho K, Meyerhardt JA, Kirkner GJ, Chan AT, Kawasaki T, et al. Cohort study of fatty acid synthase expression and patient survival in colon cancer. J Clin Oncol. 2008;26:5713–20.
Zaytseva YY, Harris JW, Mitov MI, Kim JT, Butterfield DA, Lee EY, et al. Increased expression of fatty acid synthase provides a survival advantage to colorectal cancer cells via upregulation of cellular respiration. Oncotarget. 2015;6:18891–904.
Lu X, Kambe F, Cao X, Kozaki Y, Kaji T, Ishii T, et al. 3beta-Hydroxysteroid-delta24 reductase is a hydrogen peroxide scavenger, protecting cells from oxidative stress-induced apoptosis. Endocrinology. 2008;149:3267–73.
Shen Y, Zhou J, Nie K, Cheng S, Chen Z, Wang W, et al. Oncogenic role of the SOX9-DHCR24-cholesterol biosynthesis axis in IGH-BCL2+ diffuse large B-cell lymphomas. Blood. 2022;139:73–86.
Kędzierska H, Piekiełko-Witkowska A. Splicing factors of SR and hnRNP families as regulators of apoptosis in cancer. Cancer Lett. 2017;396:53–65.
Lin JC, Tsao MF, Lin YJ. Differential impacts of alternative splicing networks on apoptosis. Int J Mol Sci. 2016;17:2097.
Download references
Acknowledgements
The authors thank Prof. Pengcheng Bu for guidance in establishing orthotopic liver metastasis mouse models.
The animal study protocol was approved by the animal research ethics committee of Tianjin Haihe hospital.
This work was supported by the National Natural Science Foundation of China (NSFC) grant 82274031 to YZ. This work was also supported by Natural Science Foundation of Tianjin grant 21JCYBJC00180, Tianjin Key Medical Discipline (Specialty) Construction Project (TJYXZDXK-044A), and Jingjinji Cooperation Project (J230038).
Author information
Authors and affiliations.
School of Pharmaceutical Science and Technology, Tianjin University, Tianjin, 300072, China
Tong Xu, Wennan Zhao, Huixiang Li, Mingzhe Zhang, Yiqing Tian, Ge Hu & Youcai Zhang
Tianjin Key Laboratory of Animal and Plant Resistance, College of Life Sciences, Tianjin Normal University, Tianjin, 300382, China
Xichuan Li & Yuan Yue
Cancer Biology Program, University of Hawaii Cancer Center, Honolulu, HI, 96813, USA
Department of Colorectal Surgery, Tianjin Union Medical Center, Tianjin, 30021, China
Leixin Jin, Zhiqiang Feng & Chunze Zhang
State Key Laboratory of Medicinal Chemical Biology, College of Pharmacy and Tianjin Key Laboratory of Molecular Drug Research, Nankai University, Tianjin, 300350, China
Xintong Dai & Changliang Shan
Tianjin Haihe Hospital, Tianjin, 300051, China
Weihua Zhang
You can also search for this author in PubMed Google Scholar
Contributions
T.X. performed most of the experiments and analyzed the data. X.L., X.W. and W.Z. provided technical support for data analysis and animal handling. T.X., L.J., Z.F. and C.Z. performed animal surgery and model establishment. H.L., M.Z., Y.T., Y.Y. and G.H. analyzed the dada. X.D. constructed patient-derived organoids, Y.Z. supervised the study and took responsibility for all experimental design. Y.Z. drafted the manuscript. T.X., C.S., W.Z., X.W. and C.Z. revised the manuscript. All authors have read and approved the manuscript.
Corresponding authors
Correspondence to Chunze Zhang or Youcai Zhang .
Ethics declarations
Ethics approval and consent to participate.
This study was approved by the Ethics Committee of Tianjin Union Medical Center in accordance with the ethical guidelines of the Declaration of Helsinki.
Consent for publication
All authors have agreed to publish this manuscript.
Competing interests
No potential conflict of interest was reported by the author(s).
Additional information
Publisher’s note.
Springer Nature remains neutral with regard to jurisdictional claims in published maps and institutional affiliations.
Supplementary Information
Additional file 1..
Supplementary materials and methods.
Additional file 2:
Table S1. Positively TCGA. Table S2. Negatively TCGA. Table S3. RNA-Seq. Table S4. Lipidomics.
Additional file 3.
Supplementary figures and tables. Figure S1. SF3B3 is upregulated in human CRC and regulated by H3K27ac. (A) SF3B7 transcript levels in normal and CRC tissues. TCGA-COAD and TCGA-READ datasets (normal, n =51; cancer, n =383) were obtained from Xena (http://xena.ucsc.edu/). Data are shown as mean ± SD. (B) mRNA levels of SF3b family members in CRC tissues from TCGA-COAD and TCGA-READ datasets in cBioPortal (https://www.cbioportal.org/). (C) mRNA levels of SF3b family members in normal and CRC tissues after integrating the data of normal colon tissues from GTEx datasets with TCGA datasets. Analysis was performed by GEPIA2 (http://gepia2.cancer-pku.cn/). (D) KEGG pathway analysis of SF3B3 positively and negatively co-expressed genes that were identified using cBioPortal (https://www.cbioportal.org/). (E) Correlation analysis between SF3B3 and MKI67 mRNA levels in TCGA datasets from Xena (http://xena.ucsc.edu/). (F) Potential epigenetic factors for SF3B3 gene using a parameter of 1-kb regulatory potential decay in Cistrome DB Toolkit (http://dbtoolkit.cistrome.org). (G) Visualization of H3K27ac enrichment in the promoter region of SF3B3 gene using UCSC Genome Browser (http://genome.ucsc.edu/). (H) Genome browser view of H3K27ac occupancy in SF3B3 promoter region. ChIP-Seq data from three CRC cell lines (GSE83968, GSE96069, and GSE71510) were retrieved from GEO datasets (http://www.ncbi.nlm.nih.gov/geo). (I) SF3B3 mRNA levels in LoVo and HT29 cells treated with 40 μM curcumin for 24 h. FOXM1 was used as positive control for H3K27ac target. Data are shown as mean ± SD. Figure S2. SF3B3 promotes proliferation and metastasis in vitro. (A) qRT-PCR quantification of SF3B3 mRNA expression as well as representative western blots of SF3B3 in 7 CRC cell lines and normal human colon mucosal epithelial cell line (NCM460). (B) qRT-PCR analysis of SF3B3 mRNA as well as representative western blots of SF3B3. LoVo and HT29 cells were transfected with siRNAs and collected at 48 h for mRNA detection or at 72 h for protein detection. SW480 cells were transfected with SF3B3 -overexpressing plasmids for 72 h. (C) Growth curves and (D) colony formation of LoVo and HT29 cells after SF3B3 knockdown by siRNAs (siNC vs siSF3B3#2), or after SF3B3 knockdown by siRNAs for 12 h followed with re-expression of SF3B3 (si SF3B3 #2+EV vs si SF3B3 #2+SF3B3) (empty vector, EV; SF3B3 overexpressing plasmid, SF3B3). (E) Growth curves and colony formation of SW480 cells after transfection with SF3B3 overexpressing plasmids. (F) Wound healing assays and (G) Transwell assays were used to measure cell migration and invasion abilities. LoVo and HT29 cells were treated with siRNAs (siNC vs si SF3B3 #2) to knockdown SF3B3 . For SF3B3 re-expression study, cells were treated with siRNAs (siNC vs si SF3B3 #2) for 12 h, followed by transfection with EV or SF3B3 overexpressing plasmids (si SF3B3 #2+EV vs si SF3B3 #2+SF3B3). Scale bars, 100 μm. (H) Statistical analysis of Wound healing and Transwell assay results. Data are shown as mean ± SD. (I) Representative immunofluorescence staining images of E-cadherin (green), VIM (red) and DAPI (blue) in CRC cells using a confocal microscope with 100× oil immersion lens. Figure S3. Silencing of SF3B3 impedes CRC proliferation and metastasis in vivo. qRT-PCR and western blot analyses of stably SF3B3 -knockdown LoVo (A) and HT29 (B) cells. LoVo cells were infected with sh SF3B3 #1 or sh SF3B3 #2 lentivirus, whereas HT29 cells were infected with sh SF3B3 #2 lentivirus. (C) Body weights of LoVo cell xenograft nude mice. The stably SF3B3 -knockdown LoVo cells (LoVo-sh SF3B3 #1) were subcutaneously injected into flank region of nude mice. (D) Representative IHC images of E-cadherin and VIM proteins in LoVo-shNC and LoVo-sh SF3B3 #1 xenografts. Scale bars, 25 μm. (E) Representative images of lung, H&E staining for lung tissues, and statistical analysis of metastatic nodules in lung ( n =5/group). Control and SF3B3 -knockdown HT29 cells (sh SF3B3 #2) were injected into nude mice via the tail vein. Scale bars, 200 μm. Data are shown as mean ± SD. (F) Representative IHC images of E-cadherin and VIM proteins in metastasis lung and liver tissues. Scale bars, 200 μm. Figure S4. SF3B3 regulates mitochondria-mediated apoptosis in CRC cells. (A) Cell viability of SF3B3 -knockdown CRC cells co-treated with apoptosis-, necrosis- or ferroptosis-inhibitors. LoVo and HT29 cells were transfected with siRNAs for 24 h, followed by treatment with 20 μM ZVF (Z-VAD-FMK), 10 μM NSA (necrosulfonamide), 1 μM Lip-1 (liproxstatin-1) or 1 μM Fer-1 (ferrostatin-1) for 48 h. (B) Cell viability of SF3B3 -knockdown CRC cells co-treated with ROS inhibitor. LoVo and HT29 cells were transfected with siRNAs for 24 h, followed by treatment with 10 mM NAC (N-acetylcysteine) for 48 h. (C) Representative western blots of apoptosis-related proteins. LoVo and HT29 cells were transfected with siRNAs for 24 h, followed by 10 mM NAC for 48 h. Data are shown as mean ± SD. Figure S5. SF3B3 regulates lipogenesis in CRC cells via SREBF1-FASN signaling. (A) Heatmap and (B) volcano plot displaying the significantly differential expression genes from RNA-seq of LoVo cells after transfection with siRNAs (mixture of si SF3B3 #1 and si SF3B3 #2) for 48 h. (C) Transcript levels of lipogenesis-related genes from RNA-seq of control and SF3B3 -knockdown LoVo cells. (D) Transcript levels of SREBF2 in RNA-seq analysis of SF3B3 -knockdown LoVo cells, as well as qRT-PCR analysis of SREBF2 mRNA in CRC cells after transfection with siRNAs for 48 h. (E) Triglyceride levels in SF3B3-knockdown CRC cells after transfection with siRNAs for 72 h. (F) Nile red staining (Red) images and analysis of SF3B3 -knockdown CRC cells after transfection with siRNAs for 72 h. Nuclei (blue) were stained with DAPI. Scale bars, 50 μm. (G) Representative fluorescence images of ROS (green) staining. CRC cells were transfected with siRNAs for 24 h, followed by treatment with 10 μM palmitate for 48 h. Scale bars, 50 μm. (H) Concentrations of individual fatty acids in LoVo cells after transfection with siRNAs (mixture of siSF3B3#1 and si SF3B3 #2) for 72 h. The data are calculated based on lipidomic study. (I) Correlation analysis of the mRNA expression between SF3B3 and lipogenesis-related genes based on TCGA-COAD and TCGA-READ datasets using GEPIA2. (J) qRT-PCR quantification of the mRNA expression of SREBF1 and its target genes. (K) Representative western blots of p-S6K, SREBF1, ACLY, and FASN. LoVo and HT29 cells were transfected with siRNAs (mixture of siSF3B3#1 and siSF3B3#2) for 12 h, followed by transfection with combined si TSC1 and si TSC2 for 60 h. Data are shown as mean ± SD. Figure S6. SF3B3 regulates mTOR splicing and autophagy. (A) mRNA levels of mTOR in SF3B3 -knockdown LoVo cells from RNA-seq data. (B) mRNA expression of mTOR was quantified by qRT-PCR in CRC cells after transfection with siRNAs for 48 h. (C) KEGG pathway enrichment analysis of SF3B3-regualted AS events from RNA-seq data. Differentially alternative splicing patterns were determined using the rMATS tool. (D) Representative western blots of mTORα and mTORβ in the protein extracts of HEK293T cells using previous reported N-terminal mTOR antibody (sc-517464). (E) Cell viability and representative western blots of mTOR and SREBF1 of SF3B3 -knockdown CRC cells after mTOR (+exon8 or -exon8) overexpression. LoVo and HT29 cells were transfected with si SF3B3 #1 for 12 h, followed by transfection with overexpressing plasmids for full length mTOR (+exon 8) or mTOR variant 3 (-exon 8) for 60 h. (F) Exon 8 skipping of mTOR was examined by 3% agarose gel electrophoresis of PCR products in SW480 cells. Representative western blots of mTORα and mTORβ in SW480 cells using the mTOR antibody (sc-517464). SW480 cells were transfected with SF3B3 -overexpressing plasmids for 72 h. Data are shown as mean ± SD. (G) RNA-IP analysis of the enrichment of mTOR in SF3B3 protein-antibody-beads system in LoVo cells. (H) Representative immunofluorescence images of LC3B (green) and DAPI (blue) in SF3B3 -knockdown CRC cells using a confocal microscope with 63× oil immersion lens. LoVo and HT29 cells were transfected with siRNAs for 72 h. (I) Representative western blots of LC3B in SF3B3 -knockdown CRC cells after forced expression of mTOR. LoVo and HT29 cells were transfected with siRNAs for 12 h, followed by transfection with mTOR-overexpressing plasmids for 60 h. (J) Representative western blots of LC3 in SF3B3 -knockdown CRC cells after treatment with autophagy inhibitors. LoVo and HT29 cells were transfected with siRNAs for 24 h, followed by treatment with 20 μM CQ or 80 nM BafA1 for 48 h. (K) mTOR isoform structures identified in TCGA datasets (illustrated by GEPIA2). Correlation analysis between the SF3B3 mRNA levels and full-length mTOR-001 based on BRCA-, CESC- and LIHC-TCGA datasets using GEPIA2. (L) Exon 8 skipping of mTOR was examined by 3% agarose gel electrophoresis of PCR products in SF3B3 -knockdown MCF7, Hela and Huh7 cells transfected with siRNAs for 48 h. (M) Representative western blots of mTOR and LC3 in SF3B3 -knockdown MCF7, Hela and Huh7 cells transfected with siRNAs for 72 h. Data are shown as mean ± SD. Figure S7. Effect of SF3B3 knockdown and mTOR inhibitor on CRC. (A) The clinical information of three donor patients investigated in this study. (B) qRT-PCR verification of SF3B3 knockdown in two PDX murine models. PDX1 models were intratumorally treated with sh SF3B3 #1 lentivirus, whereas PDX2 models were treated with sh SF3B3 #2 lentivirus. (C) Representative H&E staining and TUNEL staining (green) of two PDXs. Scale bars, 50 μm. (D) Cell viability of LoVo and HT29 cells after transfection with siRNAs for 24 h, followed by rapamycin or everolimus treatment at the indicated concentrations for 48 h. (E) Viability and qRT-PCR validation of SF3B3 -knockdown CRC tumor organoids. Digested organoids were transduced with shSF3B3 lentivirus for 6 h, followed by reconstitution in matrigel for 7 days. (F) Body weights of PDX mice. Xenograft tumors were transplanted into left and right flanks of each nude mouse to establish PDXs. Mice were intratumorally injected with shNC lentivirus on the left flank and sh SF3B3 lentivirus (mixture of sh SF3B3 #1 and sh SF3B3 #2) on the right flank. After that, mice were orally administered vehicle (40%PEG400, 5% Tween-80 and 5% DMSO) or everolimus (5 mg/kg, every two days for 3 weeks). (G) Representative H&E staining as well as immunohistochemistry images of mTOR and Ki67 in PDX3 tumor tissues. Data are shown as mean ± SD. Figure S8. SF3B3 regulates alternative splicing of DHCR24 and apoptotic genes. (A) mRNA and protein levels of DHCR24 . CRC cells were transfected with siRNAs, and collected at 48 h for qRT-PCR and at 72 h for western blots. (B) Minigene detection showed the effects of SF3B3 knockdown on DHCR24 splicing in CRC cells. The minigene contains exons 3-4 of DHCR24 . LoVo cells were transfected with DHCR24 minigene together with SF3B3 siRNAs for 48 h. (C) Detection of cell viability and (D) ROS in stably SF3B3 -knockdown CRC cells transfected with DHCR24 overexpressing plasmid for 72 h. Data are shown as mean ± SD. (E) Alternative splicing of apoptotic genes was examined by 1-3% agarose gel electrophoresis of PCR products in CRC cells. LoVo and HT29 cells were transfected with siRNAs for 48 h. Table S5. Sequences of siRNAs. Table S6. Sequences for plasmid construction. Table S7. Sequences of shRNAs. Table S8. Primer sequences for qRT-PCR or PCR. Table S9. List of antibodies used in this study.
Rights and permissions
Open Access This article is licensed under a Creative Commons Attribution 4.0 International License, which permits use, sharing, adaptation, distribution and reproduction in any medium or format, as long as you give appropriate credit to the original author(s) and the source, provide a link to the Creative Commons licence, and indicate if changes were made. The images or other third party material in this article are included in the article's Creative Commons licence, unless indicated otherwise in a credit line to the material. If material is not included in the article's Creative Commons licence and your intended use is not permitted by statutory regulation or exceeds the permitted use, you will need to obtain permission directly from the copyright holder. To view a copy of this licence, visit http://creativecommons.org/licenses/by/4.0/ . The Creative Commons Public Domain Dedication waiver ( http://creativecommons.org/publicdomain/zero/1.0/ ) applies to the data made available in this article, unless otherwise stated in a credit line to the data.
Reprints and permissions
About this article
Cite this article.
Xu, T., Li, X., Zhao, W. et al. SF3B3-regulated mTOR alternative splicing promotes colorectal cancer progression and metastasis. J Exp Clin Cancer Res 43 , 126 (2024). https://doi.org/10.1186/s13046-024-03053-4
Download citation
Received : 05 March 2024
Accepted : 18 April 2024
Published : 26 April 2024
DOI : https://doi.org/10.1186/s13046-024-03053-4
Share this article
Anyone you share the following link with will be able to read this content:
Sorry, a shareable link is not currently available for this article.
Provided by the Springer Nature SharedIt content-sharing initiative
- Alternative splicing
Journal of Experimental & Clinical Cancer Research
ISSN: 1756-9966
- Submission enquiries: Access here and click Contact Us
- General enquiries: [email protected]
- Alzheimer's disease & dementia
- Arthritis & Rheumatism
- Attention deficit disorders
- Autism spectrum disorders
- Biomedical technology
- Diseases, Conditions, Syndromes
- Endocrinology & Metabolism
- Gastroenterology
- Gerontology & Geriatrics
- Health informatics
- Inflammatory disorders
- Medical economics
- Medical research
- Medications
- Neuroscience
- Obstetrics & gynaecology
- Oncology & Cancer
- Ophthalmology
- Overweight & Obesity
- Parkinson's & Movement disorders
- Psychology & Psychiatry
- Radiology & Imaging
- Sleep disorders
- Sports medicine & Kinesiology
- Vaccination
- Breast cancer
- Cardiovascular disease
- Chronic obstructive pulmonary disease
- Colon cancer
- Coronary artery disease
- Heart attack
- Heart disease
- High blood pressure
- Kidney disease
- Lung cancer
- Multiple sclerosis
- Myocardial infarction
- Ovarian cancer
- Post traumatic stress disorder
- Rheumatoid arthritis
- Schizophrenia
- Skin cancer
- Type 2 diabetes
- Full List »
share this!
April 24, 2024
This article has been reviewed according to Science X's editorial process and policies . Editors have highlighted the following attributes while ensuring the content's credibility:
fact-checked
peer-reviewed publication
trusted source
Mini-colons advance colorectal cancer research
by Ecole Polytechnique Federale de Lausanne
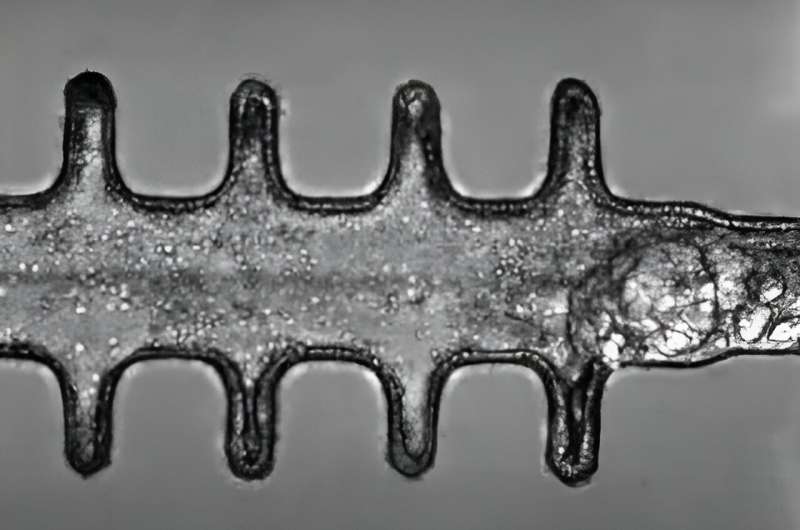
In a breakthrough for cancer research, scientists at EPFL have created lab-grown mini-colons that can accurately mimic the development of colorectal tumors, offering a powerful new tool for studying and testing treatments for the disease.
As our battle against cancer rages on, the quest for more sophisticated and realistic models to study tumor development has never been more critical. Until now, research has relied on animal models and simplified cell culture methods, which are valuable but cannot fully capture the complex interplay of factors involved in tumor development.
Even newer, more advanced models for studying cancer, such as organoids—tiny, lab-grown versions of organs—do not faithfully replicate the cell behaviors and tissue architectures seen in actual tumors.
This gap has significantly hindered our understanding of the intricate processes underlying cancer initiation, progression, and response to treatment, and calls for more sophisticated models to accurately mimic the disease's complexity.
In a significant leap forward for cancer modeling, scientists have combined microfabrication and tissue engineering techniques to develop miniature colon tissues that can simulate the complex process of tumorigenesis outside the body with high fidelity, giving rise to tumors that closely resemble those found in vivo.
The breakthrough, published in Nature , was made by Luis Francisco Lorenzo Martín, Tania Hübscher and other members of the group of Matthias Lütolf at EPFL, with input from the group of Freddy Radtke (EPFL) and colleagues at Roche's Institute of Human Biology.
The mini-colons are topobiologically complex, meaning that they not only replicate the physical structure of colon tissue, including its distinctive crypt-and-lumen architecture, but they also mimic the cellular diversity present in the actual colon tissue during healthy and diseased states.
Optogenetics: Turning cancer 'on'
Another important feature of the mini-colons is that they can be induced to develop tumors "at will" and in targeted areas—a massive advantage for cancer research . The researchers were able to turn inducible oncogenic genes on using "optogenetics." This cutting-edge technique uses light to control biological processes such as gene expression.
By integrating a blue-light-responsive system into the mini-colons, the researchers made them undergo controlled oncogenic mutations, which can reveal tumor evolution with unprecedented details. This optogenetic approach allowed the scientists to induce targeted changes in specific cell populations within the mini-colons, mimicking the localized onset of colorectal cancer in the body.
"In essence, we used light to trigger tumorigenesis by turning on oncogenic driver mutations in a spatiotemporally controlled manner in healthy bioengineered colon epithelial organoids," says Lütolf, who is also the founding director of Roche's new Institute of Human Biology.
"This basically allows you to watch tumor formation in real-time and do very detailed analyses of a process that's very difficult to study in a mouse."
The ability to trigger these genetic changes with light in the miniature colons not only allows more controlled and more precise activation of the oncogenes, but also provides a powerful tool to study the dynamic processes of tumor development and the cellular response to these mutations in real-time . This innovative use of optogenetics opens up new possibilities for dissecting the molecular and cellular mechanisms of cancer.
By manipulating genetic and environmental conditions , the researchers were also able to replicate and observe a range of tumor behaviors in the mini-colons, and even identified key factors influencing cancer progression—for example, the protein GPX2, which associated with stem cell characteristics and tumor growth.
This research offers a potent new tool for exploring the underlying mechanisms of colorectal cancer and testing potential therapies, particularly when applied to human patient-derived tissues. The mini-colons' ability to mimic tumor dynamics can reduce our reliance on animal models, which can accelerate the discovery and development of effective treatments.
Explore further
Feedback to editors

New study supports psilocybin's potential as an antidepressant

Global study reveals stark differences between females and males in disease burden causes

Researcher discusses mechanism behind a birth defect affecting brain size
3 hours ago

Study indicates that cancer patients gain important benefits from genome-matched treatments

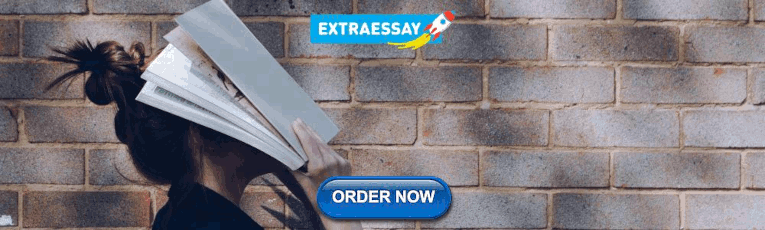
Machine learning tool identifies rare, undiagnosed immune disorders through patients' electronic health records

New technique improves T cell-based immunotherapies for solid tumors
4 hours ago

Unraveling the roles of non-coding DNA explains childhood cancer's resistance to chemotherapy

Conscious memories of childhood maltreatment strongly associated with psychopathology
5 hours ago

Study finds private equity expanding to mental health facilities

Organ transplant drug may slow Alzheimer's disease progression
Related stories.

Miniature colons with immune components aid the study of intestinal diseases
Nov 20, 2023

Transcription factor found to play pivotal role in development of right-sided colon cancers
Feb 27, 2024

Genetic switches in tumor development may lead to new therapeutic target for colorectal cancer
Nov 29, 2023

Studying the function of liver cancer genes in mini-organs
May 23, 2019

Organoids help bridge gap between laboratory study and animal modeling of colorectal cancer
Aug 5, 2020

New insights into liver cancer using organoids
May 3, 2023
Recommended for you

Preclinical study finds novel stem cell therapy boosts neural repair after cardiac arrest
6 hours ago

With huge patient dataset, AI accurately predicts treatment outcomes
7 hours ago

New mRNA cancer vaccine triggers fierce immune response to fight malignant brain tumor
9 hours ago
Let us know if there is a problem with our content
Use this form if you have come across a typo, inaccuracy or would like to send an edit request for the content on this page. For general inquiries, please use our contact form . For general feedback, use the public comments section below (please adhere to guidelines ).
Please select the most appropriate category to facilitate processing of your request
Thank you for taking time to provide your feedback to the editors.
Your feedback is important to us. However, we do not guarantee individual replies due to the high volume of messages.
E-mail the story
Your email address is used only to let the recipient know who sent the email. Neither your address nor the recipient's address will be used for any other purpose. The information you enter will appear in your e-mail message and is not retained by Medical Xpress in any form.
Newsletter sign up
Get weekly and/or daily updates delivered to your inbox. You can unsubscribe at any time and we'll never share your details to third parties.
More information Privacy policy
Donate and enjoy an ad-free experience
We keep our content available to everyone. Consider supporting Science X's mission by getting a premium account.
E-mail newsletter
- Open access
- Published: 30 April 2024
Circulating-tumour DNA methylation of HAND1 gene: a promising biomarker in early detection of colorectal cancer
- Mehrdad Shavali 1 na1 ,
- Arash Moradi 2 na1 ,
- Mohammad Tahmaseb 1 ,
- Kamal Mohammadian 3 , 4 &
- Shahla Mohammad Ganji 2
BMC Medical Genomics volume 17 , Article number: 117 ( 2024 ) Cite this article
Metrics details
Colorectal cancer (CRC) is one of the significant global health concerns with an increase in cases. Regular screening tests are crucial for early detection as it is often asymptomatic in the initial stages. Liquid biopsies, a non-invasive approach that examines biomarkers in biofluids, offer a promising future in diagnosing and screening cancer. Circulating-tumour DNA (ctDNA) is the genetic material in biofluids released into the circulatory system by cells. ctDNA is a promising marker for monitoring patients since cancer cells display distinct DNA methylation patterns compared to normal cells. The potential of our research to contribute to early detection and improved patient outcomes is significant.
The primary objective of this research project was to explore the HAND1 methylation levels in plasma ctDNA as a potential biomarker for diagnosing CRC and evaluate the methylation level of the well-established gene SPET9 to compare it with the methylation level of HAND1 .
Materials and methods
Plasma samples were collected from 30 CRC patients and 15 healthy individuals, with CRC samples obtained pre-treatment. ctDNA was extracted and treated with bisulfite for methylation status assessment. Quantitative methylation-specific PCR (qMS-PCR) was performed for HAND1 and SEPT9 , using β-actin ( ACTB gene) as a reference. The study aims to evaluate the potential of these genes as diagnostic biomarkers for CRC, contributing to early detection and improved patient outcomes.
Our study yielded significant results: 90% of CRC patients (27 out of 30) had hypermethylation in the SEPT9 gene, and 83% (25 out of 30) exhibited hypermethylation in the HAND1 gene. The methylation levels of both genes were significantly higher in CRC patients than in healthy donors. These findings underscore the potential of SEPT9 and HAND1 methylation as promising biomarkers for diagnosing CRC, potentially leading to early detection and improved patient outcomes.
These findings highlight the potential of SEPT9 and HAND1 methylation as promising biomarkers for diagnosing CRC. However, further research and validation studies are needed to confirm these findings and to explore their clinical utility in CRC diagnosis and management.
Peer Review reports
Introduction
Colorectal cancer (CRC) is a significant global health concern; unfortunately, incidence rates are anticipated to continue to rise in the coming years. In 2023, it is estimated that the United States will experience approximately 153,020 new cases of CRC and 52,550 deaths related to the disease [ 1 ]. Despite a decrease in overall incidence, there has been a concerning shift toward younger age at diagnosis, advanced stage at diagnosis, and left colon/rectum cases [ 2 ]. Regular screening tests are crucial in detecting and diagnosing CRC early, as it is often considered a “silent” disease with no noticeable symptoms in its initial stages [ 3 ]. It is possible for patients not to experience bleeding or abdominal pain until the cancer has progressed to advanced stages, which can make treatment and cure more difficult.
Preventing disease spread and minimising illness’s impact on individuals is critical. Liquid biopsies, a medical method that examines biomarkers in biofluids (including blood plasma, saliva, cerebrospinal fluid, and urine), hold great potential as a non-invasive approach to diagnosing and screening cancer [ 4 ]. Circulating-tumour DNA (ctDNA) is a genetic material present in biofluids and released into the circulatory system by cells through various mechanisms. The release of ctDNA can occur due to apoptotic, necrotic, or tumoural activity. These DNA fragments range from a few to several hundred base pairs [ 5 ]. For instance, stepwise epigenetic and genetic alterations occur during the initiation and progression of CRC. There is increasing evidence that cancer cells display distinct DNA methylation patterns compared to normal cells. Typically, DNA methylation levels are decreased within cancer cells in areas of low CpG density compared to healthy cells. Conversely, a specific subset of CpG islands exhibit hypermethylation in a cancer cell-specific manner [ 6 ]. Hence, extracting valuable genetic information from tumour ctDNA could be crucial for cancer diagnosis, treatment, and screening.
HAND1 , a gene located at chromosome 5q33, plays a crucial role in differentiating trophoblast giant cells and cardiac morphogenesis during development. Its involvement in cell proliferation and differentiation of trophoblast and cardiomyocytes has been well-documented [ 7 ]. In several types of cancer, including colorectal, pancreatic, small cell lung, ovarian, thyroid, and melanoma, HAND1 is downregulated and methylated [ 8 ]. This gene, epigenetically silenced in colon cancer, has been identified as a Polycomb target closely associated with ES cell differentiation. Intriguingly, ectopic expression of HAND1 triggers terminal differentiation and impedes the growth, proliferation, and xenograft tumour formation of colorectal cancer cells [ 9 ]. However, the underlying mechanism of this phenomenon remains a challenge, necessitating further research on HAND1 as a potential tumour suppressor in various cancer types. The possible implications of this research on cancer treatment underscore the importance of our collective efforts in this field.
ctDNA could be a promising marker for selecting and monitoring patients using the “watch and wait” approach. It is detected in about 75% of patients with CRC at the baseline and about 15–20% in the post-neoadjuvant or postoperative setting [ 10 ]. The primary objective of this research project was to explore the potential of methylation levels of well-known Septin-9 ( SEPT9 ) and introduce a novel gene, Heart and Neural Crest Derivatives Expressed 1 ( HAND1 ), in plasma ctDNA as the putative biomarker for diagnosing CRC. Specifically, this study aimed to investigate the methylation pattern of HAND1 in ctDNA plasma samples for the first time. We utilised quantitative methylation-specific real-time PCR (qMS-PCR) to evaluate the levels of hypermethylation of HAND1 and SEPT9 in ctDNA plasma samples obtained from Iranian patients diagnosed with CRC.
Bioinformatic-based study of SEPT9 and HAND1 genes methylation level based on TCGA data
The SEPT9 and HAND1 promoter methylation status was analysed in Colorectal cancer using the UALCAN database ( https://ualcan.path.uab.edu/ ). To do so, we obtained the data of Colon adenocarcinoma (COAD) patients, including Normal ( n = 37), Stage1 ( n = 50), Stage2 ( n = 122), Stage3 ( n = 88), and Stage4 ( n = 41) patients data. UALCAN is a user-friendly and interactive web resource that comprehensively analyses cancer OMICS data, such as TCGA [ 11 ].
Study design
In our previous bioinformatics-based study [ 12 ], we examined the areas where differential methylation in tissues affected by colorectal cancer (CRC). Our analysis uncovered a group of genes downregulated in CRC tissues due to hypermethylation. Within this group of genes, HAND1 was one of the genes that showed significant hypermethylation and downregulation. Consequently, in the present study, we elected to scrutinise the methylation status of two genes, specifically SEPT9 and HAND1, within the plasma of CRC patients. Our earlier discovery informed this decision about the HAND1 gene. The study design and methodology were carefully planned to ensure the reliability and validity of our findings.
Patient and sample collection
Plasma samples from 30 patients with CRC and 15 healthy individuals were obtained from those referred to Besat Hospital in Hamedan Province, Iran, during January and March 2022. Healthy donors were checked for the absence of inflammation. Also, the samples of patients with CRC were collected before starting treatment (surgery or chemotherapy). Plasma samples were stored in the liquid nitrogen at -70˚C up to date of use for further analysis. The permission to conduct the present investigation was obtained from the Ethical Committee of NIGEB (Ethical Code: IR.NIGEB.EC.1401.12.14.D), and informed consent was obtained from patients.
ctDNA extraction, bisulfite conversion, qMS-PCR
ctDNA was extracted from all the plasma samples using the standard protocol of the AddPrep Genomic DNA Extraction Kit (add Bio-Korea). Nanodrop evaluated the number of ctDNA. For assessing methylation status, EZ DNA Methylation-lightning TMKit (Zymo research-US) was used to treat 500ng of the isolated DNA with bisulfite, based on the manufacturer’s instruction.
This study aims to evaluate methylation status by Quantitative methylation-specific PCR (qMS-PCR) performed for HAND1 and SEPT9 , and β-actin ( ACTB gene) as reference genes. The specific primers for methylated and unmethylated genes are designed as listed in Table 1 . Bisulfite-treated HCT116 cell line (NCBIcode: C570) was used as the positive control, and ultrapure water was used as the control negative. The analysis of qMS-PCR was performed by utilising the MIC machine, USA, and SYBR Green reagents, which were triplicated on each sample. ACTB was selected as an endogenous reference gene in this analysis. qMS-PCR was performed using 1 µl of ctDNA (at a concentration of 50 ng/ µl), 0.3 µl of each forward and reverse primer, 6 µl of Hotstart RealQ Plus 2x Master Mix Green Without ROX (Amplicon, Denmark) and 4.4 µl of water in final of 12 µl. PCR amplification was run for these genes at two steps, with 1 of 95 °C for 5 min followed by step 2 composed of 40 cycles of 94 °C 30 s, 58 °C 45 s, and final 72 °C for 5 min extension. The analysis of methylation marker abundance of studied genes was calculated using REST 2009 software and according to the formula: 2 −(CtMarker−CtACTB) *100 .
Data analysis
Statistical analysis was performed using GraphPad Prism 9.3.1. The Kolmogorov evaluated the normality and parameters of data using the Smirnov test. The expression levels of the candidate genes and patient’s demographics and clinical features, in addition to pathological risk factors, were tested by independent-sample t -test and one-way ANOVA, including Bonferroni and Tukey HSD tests following the assessment of the homogeneity of variances through the application of Levene’s test. The 95% confidence interval was used in all tests, and the p -value < 0.05 was considered significant. Mann–Whitney Plasma methylation levels between CRC cases and controls were compared using the U test to determine statistical significance. The sensitivity and specificity of the assay were evaluated using a receiver operating characteristic (ROC) curve, and the area under the ROC curve (AUC) was determined using a non-parametric method. The sensitivity of the methylation assay was calculated by determining what percentage of stage III-IV patients tested positive, and the specificity was calculated by determining what percentage of healthy controls tested negative.
SEPT9 and HAND1 genes methylation status
The data analysis reveals an interesting finding regarding the promoter SEP9 gene, indicating a significant difference in methylation status across different stages of COAD when compared to the normal group. Specifically, the p-value of SEP9 gene expression was found to be 1.63E-12 for Stage1, <1E-12 for Stage2, 1.62E-12 for Stage3, and 1.11E-10 for Stage4 (Fig. 1 -A). Similarly, the promoter of the HAND1 gene also showed a significant methylation alteration across the stages of COAD compared to the normal group. Specifically, the p-value of HAND1 gene expression was found to be 1.85E-13 for Stage1, < 1.62E-12 for Stage2, 1.62E-12 for Stage3, and 2.43E-05 for Stage4 (Fig. 1 -B).
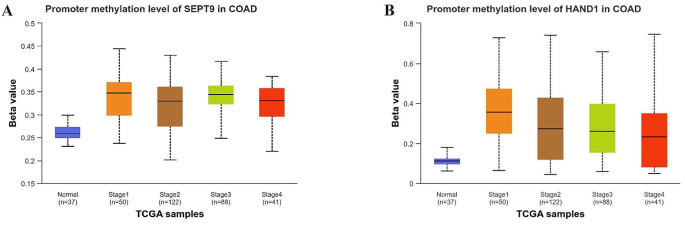
Promoter methylation levels based on TCGA data. The methylation levels of ( A ) SEPT9 and ( B ) HAND1 genes. Https://ualcan.path.uab.edu/ provides charts. COAD, Colon Adenocarcinoma
Patient and sample characteristics
The study comprised a group of patients diagnosed with CRC, consisting of 18 males (60%) and 12 females (40%), with an average age of 50.13 years and an age range of 31 to 68 years. On the other hand, the control group was composed of 8 males (54%) and 7 females (46%), with a slightly higher average age of 53.80 years and a wider age range of 29 to 72 years. The measured mean CRP levels in mg/dL were significantly higher ( p < 0.001) in the CRC group, at approximately 23.08 mg/dL, compared to the control group. The concentrations of ctDNA in blood plasma were determined by microvolume spectrophotometer Nanodrop. The results conveyed that the concentrations of ctDNA in patients with CRC range from 19.62 to 296.31 ng/µL (median 171.90 ng/µL). The range of ctDNA concentration in healthy donors was significantly ( p < 0.001) lower, from 12.68 to 126.01 ng/mL (median 58.79 ng/µL) (Table 2 ; Fig. 2 -A). Due to the limitation in sample size, there was no significant correlation among the CRP, CA19-9, and CEA levels with ctDNA concentrations.
ctDNA methylation levels of SEPT9 and HAND1 genes in plasma samples
Bisulfite treatment followed by quantitative methylation-specific polymerase chain reaction (qMS-PCR) was used to detect the methylation status of SEPT9 and HAND1 genes accurately. Our findings revealed that 90% of the samples from CRC patients (27 out of 30) had hypermethylation in the SEPT9 gene, while 83% (25 out of 30) of the CRC patients exhibited hypermethylation in the HAND1 gene. The methylation levels of the SEPT9 gene were significantly ( p = 0.009) higher in CRC patients (1.865 ± 2.053) than in healthy donors (0.0133 ± 2.591) (Fig. 2 -B, D) with a p-value of 0.009. Notably, the methylation levels of the SEPT9 CpG site in CRC patients showed a sensitivity of 66.67% (95% CI, 41.71–84.82%) and a specificity of 86.67% (95% CI, 70.32–94.69%), with a cut-off > 1.56. Similarly, the HAND1 gene’s methylation status in CRC patients (1.617 ± 2.286) was significantly ( p < 0.001) higher than in healthy donors (-1.545 ± 2.292) (Fig. 2 -C, E). The methylation levels of the HAND1 CpG site in CRC patients showed a sensitivity of 93.33% (95% CI, 70.18–99.66%) and a specificity of 80.00% (95% CI, 62.69–90.49%), with a cut-off > 1.57.
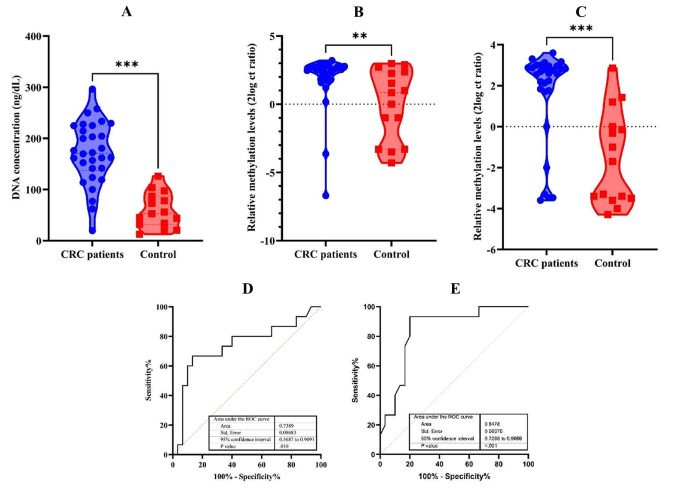
Concentration of extracted ctDNA from plasma samples and the relative methylation levels of genes. ( A ) ctDNA concentrations in CRC patients compared to normal controls. The mean ± SD of ctDNA concentrations in CRC patients was 171.90 ± 62.34, which is significantly ( p < 0.001) higher than normal controls (58.79 ± 34.07). ( B ) Relative methylation levels of SEPT9 (**, p = 0.009). ( C ) Relative methylation levels of HAND1 (***, p < 0.001). ( D ) ROC curve for SEPT9 ( E ) ROC curve for HAND1 . ROC, Receiver-operating characteristics
Tumour diagnosis is conventionally carried out by examining tissues through biopsy, considered the most reliable method. However, there are several limitations to this approach. For example, it is ineffective in detecting early-stage tumours or residual lesions. Moreover, this technique has limited application in assessing the effectiveness of treatment and predicting the prognosis of the disease. One promising biomarker that can be used to detect malignancy is circulating tumour DNA (ctDNA) found in the blood plasma. This biomarker can help overcome the limitations of tissue biopsy-based diagnosis and enable a more accurate and comprehensive cancer diagnosis [ 13 ]. Studies have shown that changes in ctDNA concentration can be linked to cancer patients’ development, prognosis, and survival [ 14 ]. The concentration of ctDNA tends to increase in patients with breast, gastric, ovarian, lung, colon, and prostate cancer, suggesting that it is associated with apoptosis and necrosis of cancer cells in the tumour microenvironment [ 15 ]. Researchers have also found specific cancer-related alterations in blood ctDNA, such as allelic imbalances, methylation, and mutations, which support this theory [ 16 ].
This study found that the concentration range of ctDNA in patients with CRC was between 19.62 and 296.31 ng/µL, with a median of 171.90 ng/µL. The concentration of ctDNA in healthy donors was significantly lower ( p < 0.001), ranging from 12.68 to 126.01 ng/mL, with a median of 58.79 ng/µL. Previous research has revealed that the concentration of ctDNA in CRC patients can vary depending on the cancer stage and detection methods used. The range has been reported to be between 10 and 1000 ng/µL in some studies [ 17 ], while others have shown it to be between 0.1 and 12 ng/µL. Similarly, the ctDNA concentration in healthy donors has also varied in past studies, ranging from 0 to 100 ng/µL [ 18 ]. It is important to note that comparing results from different studies can be challenging due to differences in sample size, patient populations, and detection methods. Thus, elevated levels of ctDNA in patients’ plasma could be a biomarker for the presence of malignancy.
Recent studies have identified that the methylation of CPG islands in gene promoters, particularly SEPT9 , could be used as a promising DNA biomarker for CRC diagnosis. SEPT9 is a member of the conserved family of cytoskeletal GTPases and is involved in various biological processes such as cytokinesis, polarisation, vesicle trafficking, membrane reconstruction, DNA repair, cell migration, and apoptosis [ 19 ]. Studies have shown that the SEPT9 gene is regulated by a CpG island in its promoter region [ 19 ]. Methylation of the Spetin9 gene ( m SEPT9 ) is the most extensively studied biomarker in CRC recurrence, and it is the only methylation-based biomarker approved for CRC diagnosis by the Food and Drug Administration (FDA) [ 20 ]. The methylated SEPT9 ( m SEPT9 ) has shown promise as a circulating biomarker for detecting colorectal cancer. In this study, we found that the methylation levels of SPET9 were significantly ( p = 0.009) higher in CRC patients with a sensitivity of 66.67% (with a 95% confidence interval from 41.71 to 84.82%) and a specificity of 86.67% (with a 95% confidence interval from 70.32 to 94.69%), using a cut-off value of higher than 1.56. Aligned with our study, an investigation on ctDNA in CRC patients found that m SEPT9 was positive in 73% of CRC patients at 94.5% specificity. The sensitivity and specificity of m SEPT9 for diagnosis and recurrence monitoring were higher than that of CEA, CA19–9, and CA724 [ 21 ].
Furthermore, the methylation status of the HAND1 gene in CRC patients was significantly higher (1.617 ± 2.286) compared to healthy donors (-1.545 ± 2.292) with a p-value of less than 0.001. Our study revealed that the methylation levels of the HAND1 CpG site in CRC patients demonstrated a sensitivity of 93.33% (95% CI, 70.18–99.66%) and a specificity of 80.00% (95% CI, 62.69–90.49%), with a cut-off value greater than 1.57. It has been demonstrated that the expression of HAND1 is controlled by the promoter region, which is regulated by cytosine methylation [ 8 ]. HAND1 /2 proteins are essential in heart development, promoting cell proliferation and differentiation alongside other transcription factors like Nkx2-5 and GATA4. Researchers are interested in HAND1 /2’s possible role as a tumour suppressor and how its downregulation may contribute to cardiomyopathy development. It is crucial to comprehend the multifaceted roles of these transcription factors in normal heart development and tumour formation [ 22 ]. Furthermore, studies demonstrated that the HAND1 gene was hypermethylated and downregulated in gastric cancer [ 8 ] and is believed to function as a tumour suppressor gene. These findings suggest that HAND1 gene methylation status may be a potential diagnostic biomarker for CRC patients.
In this study, we assessed the potential clinical usefulness of the SPET9 and HAND1 biomarkers by calculating their sensitivity and specificity. The bisulfite treatment followed by qMS-PCR was used to detect the methylation status of genes, which enhanced the study’s reliability. Nevertheless, the study had some limitations that require further attention. For example, the limited sample size must be expanded to achieve more statistically meaningful outcomes. Further longitudinal data on ctDNA concentrations or methylation status variations concerning disease progression or treatment response should be undertaken. On the other hand, there is a recommendation to conduct more investigations on dimension reduction approaches [ 23 ], which are critical to enhancing cancer research. This method can facilitate the selection of the most informative potential biomarkers that could impact the diagnostic precision. It is suggested that examining methylation alterations in non-cancerous conditions like polyps and inflammatory bowel disease (IBD) can differentiate between malignant and non-malignant diseases. Therefore, more research is crucial to validate these findings and comprehend their practicality in a clinical setting.
In conclusion, the study’s findings provide valuable insights into the potential of SEPT9 and HAND1 methylation as highly promising biomarkers for diagnosing CRC. These epigenetic alterations have been shown to exhibit high sensitivity and specificity in differentiating CRC from non-cancerous tissues and other types of colorectal cancer. However, it is essential to note that further research and validation studies are necessary to corroborate these results and to assess their clinical usefulness in CRC diagnosis and management. The development of reliable biomarkers for CRC detection is crucial for improving early diagnosis and treatment outcomes, and it is hoped that the findings of this study will pave the way for more effective diagnostic tools and therapeutic strategies in the future.
Data availability
The data generated and/or analysed during the current study are not publicly available but are available from the corresponding author who organised the study.
Siegel RL et al. Colorectal cancer statistics, 2023 CA: A Cancer Journal for Clinicians, 2023. 73(3): pp. 233–254.
Stoffel EM, Murphy CC. Epidemiology and mechanisms of the increasing incidence of Colon and rectal cancers in young adults. Gastroenterology. 2020;158(2):341–53.
Article PubMed Google Scholar
Shah R, et al. Biomarkers for early detection of Colorectal Cancer and polyps: systematic review. Cancer Epidemiol Biomarkers Prev. 2014;23(9):1712–28.
Article CAS PubMed Google Scholar
Brock G, et al. Liquid biopsy for cancer screening, patient stratification and monitoring. Translational Cancer Res. 2015;4(3):280–90.
CAS Google Scholar
Connal S, et al. Liquid biopsies: the future of cancer early detection. J Transl Med. 2023;21(1):118.
Article PubMed PubMed Central Google Scholar
Luo B, et al. Ultrasensitive DNA methylation ratio detection based on the Target-Induced nanoparticle-coupling and site-specific base oxidation damage for Colorectal Cancer. Anal Chem. 2022;94(16):6261–70.
Riley P, Anson-Cartwright L, Cross JC. The Hand1 bHLH transcription factor is essential for placentation and cardiac morphogenesis. Nat Genet. 1998;18(3):271–5.
Kuang Y, et al. The developmental regulator HAND1 inhibits gastric carcinogenesis through enhancing ER stress apoptosis via targeting CHOP and BAK which is augmented by cisplatin. Int J Biol Sci. 2023;19(1):120–36.
Article CAS PubMed PubMed Central Google Scholar
Tan J, et al. Integrative epigenome analysis identifies a polycomb-targeted differentiation program as a tumor-suppressor event epigenetically inactivated in colorectal cancer. Cell Death Dis. 2014;5(7):e1324.
Piercey O, Tie J. Circulating tumour DNA in the evolving treatment landscape of locally advanced rectal cancer: where does it fit in? Ther Adv Med Oncol. 2023;15:17588359231160138.
Chandrashekar DS, et al. UALCAN: an update to the integrated cancer data analysis platform. Neoplasia. 2022;25:18–27.
Moradi A, et al. Consequences of aberrated DNA methylation in Colon adenocarcinoma: a bioinformatic-based multi-approach. BMC Genom Data. 2022;23(1):83.
Hirahata T, et al. Liquid Biopsy: A Distinctive Approach to the diagnosis and prognosis of Cancer. Cancer Inf. 2022;21:11769351221076062.
Google Scholar
Khurram I, et al. Efficacy of cell-free DNA as a diagnostic biomarker in breast cancer patients. Sci Rep. 2023;13(1):15347.
Gao Q, et al. Circulating cell-free DNA for cancer early detection. Innov (Camb). 2022;3(4):100259.
Tivey A, et al. Circulating tumour DNA — looking beyond the blood. Nat Reviews Clin Oncol. 2022;19(9):600–12.
Article CAS Google Scholar
Cha Y, Kim S, Han SW. Utilizing plasma circulating tumor DNA sequencing for Precision Medicine in the management of solid cancers. Cancer Res Treat. 2023;55(2):367–84.
Mayer S et al. Rescue of non-informative circulating tumor DNA to monitor the Mutational Landscape in NSCLC. Cancers (Basel), 2020. 12(7).
Sun J, et al. Structure and function of Septin 9 and its role in human malignant tumors. World J Gastrointest Oncol. 2020;12(6):619–31.
Potter NT, et al. Validation of a real-time PCR-based qualitative assay for the detection of methylated SEPT9 DNA in human plasma. Clin Chem. 2014;60(9):1183–91.
Sun J, et al. The role of mSEPT9 in screening, diagnosis, and recurrence monitoring of colorectal cancer. BMC Cancer. 2019;19(1):450.
Shafi O, Siddiqui G, Jaffry HA. The benign nature and rare occurrence of cardiac myxoma as a possible consequence of the limited cardiac proliferative/ regenerative potential: a systematic review. BMC Cancer. 2023;23(1):1245.
Mukherjee S et al. In Silico Integration of Transcriptome and Interactome predicts an ETP-ALL-Specific transcriptional footprint that decodes its Developmental Propensity. Front Cell Dev Biology, 2022. 10.
Download references
Acknowledgements
The authors appreciated the Besat Hospital in Hamedan Province, Iran, for providing the present study with blood and plasma samples. Also, we would like to thank the NIGEB for providing the equipment and materials for this research. Moreover, the authors thank the Biotechnology Found Foundation (www.BioTechFund.ir) and TritaGene Biotech Co. (https://tritagene.com/en/) for supporting the present project.
Biotechnology Found Foundation with grant number 01/16204.
Author information
Mehrdad Shavali and Arash Moradi contributed equally to this work.
Authors and Affiliations
Department of Cell and Molecular Biology, Faculty of Biological Science, Kharazmi University, Tehran, Iran
Mehrdad Shavali & Mohammad Tahmaseb
Department of Medical Biotechnology, National Institute of Genetic Engineering and Biotechnology, Shahrak-e Pajoohesh, km 15, P.O. Box 14965/161, Tehran, Tehran - Karaj Highway, Iran
Arash Moradi & Shahla Mohammad Ganji
Department of Radiation Oncology, Hamadan University of Medical Sciences, Hamadan, Iran
Kamal Mohammadian
Cancer Research Center, Hamadan University of Medical Sciences, Hamadan, Iran
You can also search for this author in PubMed Google Scholar
Contributions
Arash Moradi and Shahla Mohammad Ganji designed the concepts and methodology. Mehrdad Shavali conducted experimental laboratory work. Mehrdad Shavali and Shahla Mohammad Ganji performed the data acquisition. Arash Moradi carried out bioinformatic studies, analysis and interpretation of raw data, and data visualization. Arash Moradi and Shahla Mohammad Ganji performed the manuscript writing and revisions. Mohammad Tahmaseb, Kamal Mohammadian, and Shahla Mohammad Ganji reviewed the final version of the manuscript. Shahla Mohammad Ganji provided administrative, technical, and material support. Shahla Mohammad Ganji supervised the study. All authors read and approved the final manuscript.
Corresponding author
Correspondence to Shahla Mohammad Ganji .
Ethics declarations
Ethical approval and consent to participation.
The study underwent a thorough ethical review process by the Ethical Committee of the National Institute of Genetic Engineering and Biotechnology (NIGEB) before receiving approval (Ethical code #: IR.NIGEB.EC.1401.12.14.D). The research team diligently followed all relevant guidelines and regulations to ensure the safety and well-being of all participants. Participants were given a detailed explanation of the research’s purpose and procedures and asked to sign an informed consent form before participating. The consent form described how their clinical samples and personal data would be used throughout the study, which would be conducted under their physician’s supervision. The research team created the informed consent form with great care, and all participants willingly signed it before participating. Participants were free to withdraw their consent at any point during the study. Throughout the study, the research team maintained the highest ethical standards and strictly adhere to all relevant regulations to safeguard the privacy and confidentiality of all participants’ data.
Consent for publication
Not applicable.
Competing interests
The authors declare no competing interests.
Additional information
Publisher’s note.
Springer Nature remains neutral with regard to jurisdictional claims in published maps and institutional affiliations.
Rights and permissions
Open Access This article is licensed under a Creative Commons Attribution 4.0 International License, which permits use, sharing, adaptation, distribution and reproduction in any medium or format, as long as you give appropriate credit to the original author(s) and the source, provide a link to the Creative Commons licence, and indicate if changes were made. The images or other third party material in this article are included in the article’s Creative Commons licence, unless indicated otherwise in a credit line to the material. If material is not included in the article’s Creative Commons licence and your intended use is not permitted by statutory regulation or exceeds the permitted use, you will need to obtain permission directly from the copyright holder. To view a copy of this licence, visit http://creativecommons.org/licenses/by/4.0/ . The Creative Commons Public Domain Dedication waiver ( http://creativecommons.org/publicdomain/zero/1.0/ ) applies to the data made available in this article, unless otherwise stated in a credit line to the data.
Reprints and permissions
About this article
Cite this article.
Shavali, M., Moradi, A., Tahmaseb, M. et al. Circulating-tumour DNA methylation of HAND1 gene: a promising biomarker in early detection of colorectal cancer. BMC Med Genomics 17 , 117 (2024). https://doi.org/10.1186/s12920-024-01893-9
Download citation
Received : 14 January 2024
Accepted : 25 April 2024
Published : 30 April 2024
DOI : https://doi.org/10.1186/s12920-024-01893-9
Share this article
Anyone you share the following link with will be able to read this content:
Sorry, a shareable link is not currently available for this article.
Provided by the Springer Nature SharedIt content-sharing initiative
- Methylation
- Colorectal Cancer
BMC Medical Genomics
ISSN: 1755-8794
- Submission enquiries: [email protected]
- General enquiries: [email protected]
Thank you for visiting nature.com. You are using a browser version with limited support for CSS. To obtain the best experience, we recommend you use a more up to date browser (or turn off compatibility mode in Internet Explorer). In the meantime, to ensure continued support, we are displaying the site without styles and JavaScript.
- View all journals
- Explore content
- About the journal
- Publish with us
- Sign up for alerts
- Year in Review
- Published: 08 December 2023
Colorectal cancer in 2023
New options for late-line treatment of metastatic colorectal cancer
- Sara Lonardi ORCID: orcid.org/0000-0002-7593-8138 1 &
- Filippo Pietrantonio ORCID: orcid.org/0000-0002-8530-8420 2
Nature Reviews Gastroenterology & Hepatology volume 21 , pages 76–77 ( 2024 ) Cite this article
881 Accesses
2 Citations
11 Altmetric
Metrics details
- Chemotherapy
- Colorectal cancer
Metastatic colorectal cancer is a heterogeneous disease associated with poor patient outcomes. Although the past decade has seen few first-line treatment advances, key studies published in 2023 established new options for late-line therapy of the disease with and without oncogenic drivers, thus expanding the continuum of care in metastatic colorectal cancer.
Key advances
In the SUNLIGHT trial, the addition of bevacizumab to third-line trifluridine–tipiracil (FTD/TPI) resulted in longer overall survival independent from previous exposure to VEGF inhibitors in patients with metastatic colorectal cancer (mCRC) 4 .
The FRESCO-2 study established fruquintinib as a new option for late-line treatment in patients with mCRC after failure of standard therapies including FTD/TPI and/or regorafenib 5 .
In the CodeBreaK 300 trial, the KRAS-G12C inhibitor sotorasib combined with panitumumab was superior to either FTD/TPI or regorafenib in a population of patients with pretreated KRAS G12C -mutated mCRC 10 .
This is a preview of subscription content, access via your institution
Access options
Access Nature and 54 other Nature Portfolio journals
Get Nature+, our best-value online-access subscription
24,99 € / 30 days
cancel any time
Subscribe to this journal
Receive 12 print issues and online access
195,33 € per year
only 16,28 € per issue
Buy this article
- Purchase on Springer Link
- Instant access to full article PDF
Prices may be subject to local taxes which are calculated during checkout
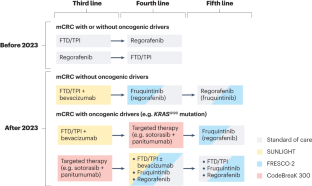
Cervantes, A. et al. Metastatic colorectal cancer: ESMO Clinical Practice Guideline for diagnosis, treatment and follow-up. Ann. Oncol. 34 , 10–32 (2023).
Article CAS PubMed Google Scholar
Grothey, A. et al. Regorafenib monotherapy for previously treated metastatic colorectal cancer (CORRECT): an international, multicentre, randomised, placebo-controlled, phase 3 trial. Lancet 381 , 303–312 (2013).
Mayer, R. J. et al. Randomized trial of TAS-102 for refractory metastatic colorectal cancer. N. Engl. J. Med. 372 , 1909–1919 (2015).
Article PubMed Google Scholar
Prager, G. W. et al. Trifluridine-tipiracil and bevacizumab in refractory metastatic colorectal cancer. N. Engl. J. Med. 388 , 1657–1667 (2023).
Dasari, A. et al. Fruquintinib versus placebo in patients with refractory metastatic colorectal cancer (FRESCO-2): an international, multicentre, randomised, double-blind, phase 3 study. Lancet 402 , 41–53 (2023).
Kopetz, S. et al. Encorafenib, binimetinib, and cetuximab in BRAF V600E-mutated colorectal cancer. N. Engl. J. Med. 381 , 1632–1643 (2019).
Yaeger, R. et al. Adagrasib with or without cetuximab in colorectal cancer with mutated KRAS G12C. N. Engl. J. Med. 388 , 44–54 (2023).
Strickler, J. H. et al. Tucatinib plus trastuzumab for chemotherapy-refractory, HER2-positive, RAS wild-type unresectable or metastatic colorectal cancer (MOUNTAINEER): a multicentre, open-label, phase 2 study. Lancet Oncol. 24 , 496–508 (2023).
Sartore-Bianchi, A. et al. Circulating tumor DNA to guide rechallenge with panitumumab in metastatic colorectal cancer: the phase 2 CHRONOS trial. Nat. Med. 28 , 1612–1618 (2022).
Article CAS PubMed PubMed Central Google Scholar
Fakih, M. G. et al. Sotorasib plus panitumumab in refractory colorectal cancer with mutated KRAS G12C. N. Engl. J. Med. https://doi.org/10.1056/NEJMoa2308795 (2023).
Download references
Author information
Authors and affiliations.
Oncology Department, Veneto Institute of Oncology IOV–IRCCS, Padua, Italy
Sara Lonardi
Medical Oncology Department, Fondazione IRCCS Istituto Nazionale dei Tumori, Milan, Italy
Filippo Pietrantonio
You can also search for this author in PubMed Google Scholar
Corresponding author
Correspondence to Sara Lonardi .
Ethics declarations
Competing interests.
S.L. reports research funding (to institution) from Amgen, Astellas, AstraZeneca, Bayer, Bristol-Myers Squibb, Daichii Sankyo, Hutchinson, Incyte, Merck Serono, Mirati, MSD, Pfizer, Roche and Servier; personal honoraria as an invited speaker from Amgen, Bristol-Myers Squibb, Incyte, GSK, Lilly, Merck Serono, MSD, Pierre-Fabre, Roche and Servier; and participation in advisory board for Amgen, Astellas, AstraZeneca, Bayer, Bristol-Myers Squibb, Daiichi-Sankyo, GSK, Incyte, Lilly, Merck Serono, MSD, Servier and Takeda. F.P. reports research funding (to institution) from Lilly, BMS, AstraZeneca, Incyte, Amgen and Agenus; personal honoraria as an invited speaker from Amgen, Merck-Serono, BMS, Servier, Bayer, Pierre-Fabre, Takeda, Ipsen and Astellas; and participation in advisory board for Amgen, Merck-Serono, MSD, Bayer, Organon, Astellas, Takeda, Servier and GSK.
Rights and permissions
Reprints and permissions
About this article
Cite this article.
Lonardi, S., Pietrantonio, F. New options for late-line treatment of metastatic colorectal cancer. Nat Rev Gastroenterol Hepatol 21 , 76–77 (2024). https://doi.org/10.1038/s41575-023-00881-1
Download citation
Published : 08 December 2023
Issue Date : February 2024
DOI : https://doi.org/10.1038/s41575-023-00881-1
Share this article
Anyone you share the following link with will be able to read this content:
Sorry, a shareable link is not currently available for this article.
Provided by the Springer Nature SharedIt content-sharing initiative
Quick links
- Explore articles by subject
- Guide to authors
- Editorial policies
Sign up for the Nature Briefing newsletter — what matters in science, free to your inbox daily.

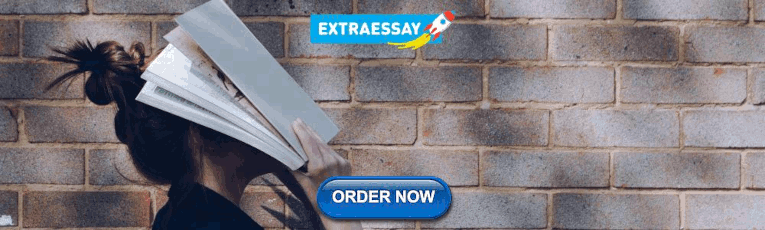
IMAGES
COMMENTS
1. Introduction. Colorectal cancer (CRC), which comprises colon and/or rectum cancer, represents a significant health problem as the world's third most commonly diagnosed and second most fatal cancer globally [].Approximately 9.4% of cancer-related deaths were due to CRC in 2020 [].However, in light of the significant increase in the number of identified cases in the older population, it is ...
According to the World Cancer Research Fund/American Institute for Cancer Research , the high consumption of dairy products (in particular milk) is probably inversely associated with the risk of developing colorectal cancer. The suggested protective effect of dairy products has been largely attributed to their content of calcium.
Colorectal cancer is one of the most common cancers worldwide. 1 In the United States, 147,000 individuals received a diagnosis of the disease in 2020, and 53,200 died from it. 2 Most patients with colorectal cancer are older than 50 years of age at diagnosis. 2 Men have a higher risk than do women and are on average 5 to 10 years younger than women when they receive the diagnosis. 3,4
Researchers have identified four warning signs that they believe may help identify colorectal cancer early in younger adults. The signs or symptoms are abdominal pain, rectal bleeding, diarrhea, and iron deficiency anemia. Drug Regimen Boosts Survival of People with Advanced Colorectal Cancer. Posted: May 24, 2023.
Colorectal cancer articles from across Nature Portfolio. Colorectal cancer, also known as bowel cancer, is a cancer formed by uncontrolled cell growth in the colon or rectum (parts of the large ...
Lung cancer is still the leading cause of cancer death (18%) followed by colorectal cancer (9.4%) . Trends in different regions of the world The number of people diagnosed with colon and rectal cancer doubled from 1990 to 2013, with most of the increase resulting from an aging and growing population.
This systematic review and meta-analysis aimed to evaluate existing evidence on the relationship between diagnostic and treatment intervals and outcomes for colorectal cancer. Four databases were ...
Advances in Colorectal Cancer Research. Colorectal cells grown into organoids, stem cell-derived human 'mini-organs' that are used to study human development and disease. NCI-funded researchers are working to advance our understanding of how to prevent, detect, and treat colorectal cancer. They are also looking at what factors influence ...
Colorectal cancer screening uptake and adherence by modality at a large tertiary care center in the United States: a retrospective analysis, Current Medical Research and Opinion, 40, 3, (431-439 ...
The incidence of early-onset colorectal cancer (in patients <50 years old) is increasing at an alarming rate. This Review highlights potential risk factors and putative mechanisms that drive this ...
7. Early detection. In a systematic review of 55 studies in a total of. 6425 patients younger than 40 years, O'Connell and colleagues, 8. reported that most common presenting symptoms in early-onset colorectal cancer were abdominal pain (55%), rectal bleeding (46%), weight loss (35%), and change in bowel habits (32%).
Abstract. Colorectal cancer (CRC) is the third most common cancer worldwide, and surgical treatment remains the first-line treatment to provide a cure. In addition to the aging population, obesity, low physical activity, and smoking habits increase CRC risk. Despite advances in surgical techniques, chemotherapy, and radiotherapy, colorectal ...
1. Introduction. Colorectal cancer (CRC) is the third most frequently detected malignancy. According to the American Cancer Society (ACS) Report 2023, the CRC is predicted to be ∼ 153,020 and a mortality rate of ∼ 52,550 (Siegel et al., 2023).The 5-year CRC patient's existence rate is nearly 90 % for those diagnosed in early (stage I & stage II) and localized stages.
Colorectal cancer (CRC) is the third most common diagnosis and second deadliest malignancy for both sexes combined. CRC has both strong environmental associations and genetic risk factors. The incidence of new cases and mortality has been steadily declining for the past years, except for younger adults (younger than 50 years), possibly related to an increase in cancer screening and better ...
Clinical Colorectal Cancer is devoted to manuscripts that focus on early detection/screening, diagnosis, prevention, and treatment of colorectal cancer and other GI cancers, including pancreatic, liver, gastric/gastroesophageal, biliary, and other gastrointestinal cancers. The major emphasis is on recent scientific developments and original peer-reviewed manuscripts.
1 Introduction. Colorectal cancer (CRC) is the second leading cause of cancer mortality in the United States ().The incidence of CRC has steadily declined since the 1980s, largely attributed to greater uptake of colonoscopy screening by adults aged 50 years and older ().Concurrently, the incidence of sporadic early-onset CRC, generally defined as CRC diagnosis before age 50 without an ...
Read the latest Research articles in Colorectal cancer from Scientific Reports. ... Colorectal cancer articles within Scientific Reports. Featured. Article 26 March 2024 | Open Access.
Consequently, colorectal cancer is a major research priority for government, pharmaceutical companies and non-profit organizations. Research into diagnosis and optimum treatment of the disease is progressing rapidly, with new advances reported every day. Colorectal Cancer presents reviews, analysis and commentary. on all aspects of colorectal ...
The DRRD was inversely related to colorectal cancer risk. The ORs of colorectal cancer were 0.77 (95% CI, 0.67-0.89) for the third versus first score tertile (P trend < 0.001) and 0.92 (95% CI, 0.87-0.96) for a 3-point increment in the score. Inverse associations were observed for colon and rectal cancers and were consistent in strata of ...
Aberrant alternative splicing (AS) is a pervasive event during colorectal cancer (CRC) development. SF3B3 is a splicing factor component of U2 small nuclear ribonucleoproteins which are crucial for early stages of spliceosome assembly. The role of SF3B3 in CRC remains unknown. SF3B3 expression in human CRCs was analyzed using publicly available CRC datasets, immunohistochemistry, qRT-PCR, and ...
Colorectal cancer (CRC) is the third most common cancer and the second most common cause of cancer deaths worldwide. The International Agency for Research on Cancer estimated that there were 1.93 million new cases of CRC and 935000 deaths from CRC in 2020 [ 1 ]. Early-onset CRC (EOCRC), largely defined as CRC occurring in adults younger than 50 ...
Important colorectal cancer (CRC) studies in 2021, including a new standard of care for first-line treatment of MSI-H-dMMR metastatic CRC, single-cell and spatial analysis of primary tumours and ...
This research offers a potent new tool for exploring the underlying mechanisms of colorectal cancer and testing potential therapies, particularly when applied to human patient-derived tissues.
This review article contains a concise consideration of genetic and environmental risk factors for colorectal cancer. Known risk factors associated with colorectal cancer include familial and hereditary factors and lifestyle-related and ecological factors. Lifestyle factors are significant because of the potential for improving our understanding of the disease. Physical inactivity, obesity ...
Background Colorectal cancer (CRC) is one of the significant global health concerns with an increase in cases. Regular screening tests are crucial for early detection as it is often asymptomatic in the initial stages. Liquid biopsies, a non-invasive approach that examines biomarkers in biofluids, offer a promising future in diagnosing and screening cancer. Circulating-tumour DNA (ctDNA) is the ...
In 2012, 614,000 women (9.2% of all new cancer cases) and 746,000 men (10.0% of new cancer cases) were diagnosed with colorectal cancer worldwide 10. Combined, in both sexes, colorectal cancer is the third-most common cancer and accounts for 9.7% of all cancers excluding non-melanoma skin cancer. More than half of the cases occur in more ...
Colorectal cancer research is eligible for funding through the military's Peer Reviewed Cancer Research Program, where it must compete against dozens of other cancers for a limited amount of ...
A genetic lineage-tracing system in human colorectal organoids identifies a population of dormant cancer cells that persists during chemotherapy and enables cancer regrowth, and the cell-adhesion ...
Inflammation and ulcers caused by Helicobacter pylori may progress to stomach cancer, and Fusobacterium nucleatum and Enterococcus faecalis have been shown to contribute to colorectal cancer ...
Metastatic colorectal cancer is a heterogeneous disease associated with poor patient outcomes. Although the past decade has seen few first-line treatment advances, key studies published in 2023 ...