An official website of the United States government
Official websites use .gov A .gov website belongs to an official government organization in the United States.
Secure .gov websites use HTTPS A lock ( Lock Locked padlock icon ) or https:// means you've safely connected to the .gov website. Share sensitive information only on official, secure websites.
- Publications
- Account settings
- Advanced Search
- Journal List
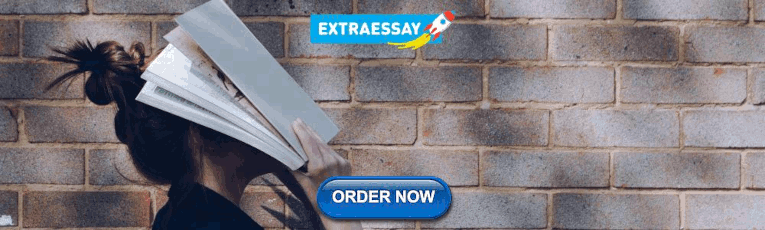
A critical review of workplace drug testing methods for old and new psychoactive substances: Gaps, advances, and perspectives
Ahmed ibrahim al-asmari.
- Author information
- Article notes
- Copyright and License information
Issue date 2024 May.
This is an open access article under the CC BY-NC-ND license (http://creativecommons.org/licenses/by-nc-nd/4.0/).
Workplace drug testing (WDT) is essential to prevent drug abuse disorders among the workforce because it can impair work performance and safety. However, WDT is limited by many challenges, such as urine adulteration, specimen selection, and new psychoactive substances (NPS). This review examined the issues related to WDT. Various scientific databases were searched for articles on WDT for drug detection published between 1986 (when WDT started) and January 2024. The review discussed the history, importance, and challenges of WDT, such as time of specimen collection/testing, specimen adulteration, interference in drug testing, and detection of NPS. It evaluated the best methods to detect NPS in forensic laboratories. Moreover, it compared different techniques that can enhance WDT, such as immunoassays, targeted mass spectrometry, and nontargeted mass spectrometry. These techniques can be used to screen for known and unknown drugs and metabolites in biological samples. This review assessed the strengths and weaknesses of such techniques, such as their validation, identification, library search, and reference standards. Furthermore, this review contrasted the benefits and drawbacks of different specimens for WDT and discussed studies that have applied these techniques for WDT. WDT remains the best approach for preventing drug abuse in the workplace, despite the challenges posed by NPS and limitations of the screening methods. Nontargeted techniques using high-resolution liquid chromatography-mass spectrometry (MS)/gas chromatography–tandem MS can improve the detection and identification of drugs during WDT and provide useful information regarding the prevalence, trends, and toxicity of both traditional and NPS drugs. Finally, this review suggested that WDT can be improved by using a combination of techniques, multiple specimens, and online library searches in case of new NPS as well as by updating the methods and databases to include new NPS and metabolites as they emerge. To the best of the author's knowledge, this is the first review to address NPS as an issue in WDT and its application and propose the best methods to detect these substances in the workplace environment.
Keywords: Workplace drug testing, Nontargeted Screening, Drug of Abuse, New psychoactive substances, High Resolution Mass Spectrometry, Low Resolution Mass Spectrometry
1. Introduction
Throughout its history, drug abuse analysis has been a necessary means of saving human lives and securing their abilities to perform well in their workplace. The side effects of illicit drug use can affect social life, workplace performance, and public safety; for example, the crime rates have increased because of the misuse of drugs. In addition to rising public concerns regarding the harmful effects and consequences of drug abuse and increases in relevant emergency department visits caused by overdoses ( Kim et al., 2019 , Els et al., 2020 , Fu et al., 2019 , White et al., 2023 ), well-trained personnel are at risk if they start using such drugs of abuse. This will markedly damage their productivity and may increase the incidence of accidents at the workplace. Therefore, companies and industries conduct workplace drug testing (WDT) to screen job applicants (pre-employment testing, random testing, and testing in cases of unjustified incidents at work), thereby achieving the growing demand for drug-free work environments in public and private sectors [5–8]. Conversely, the use of adulteration products to pass WDT examination has increased ( Embers et al., 2019 ). The desire to manipulate WDT has threatened the reliability of drug testing, and in some cases, the applicants successful to pass the WDT ( Matriciani et al., 2018 , Franz et al., 2022 , The Committee Clinical Toxicology, 2005 ). The accuracy of drug testing protocols has often been questioned, because various chemicals can be used to manipulate urine samples and cause false-negative results in WDT ( Mikkelsen and Ash, 1988 , Fu, 2019 )[13]. This study examined the different viewpoints obtained through these analyses. The approaches that have been used to detect adulteration were evaluated, and some solutions were suggested. The argument in favor of suitable detection methods to avoid false-negative results is persuasive .
Currently, drug abuse testing has become mandatory before securing certain job positions, depending on specific roles or industries. For example, this requirement applies to positions in the army and pilot roles. Drug misuse is a major factor contributing to the increase in crime rates, violence, and accidents. The National Survey on Drug Use and Health, conducted in the USA in 2015, concluded that 70 % of adults self-reporting that they have used drugs of abuse were employees, with ∼ 20 % of them being classified as having drug abuse disorder ( Els et al., 2020 ). In the USA, in 2018, the annual rate of positive drug results among employees soared to the highest level (4.4 %) among the > 9 million samples tested ( Secaucus, 2019 ). Therefore, testing for illicit drugs has a significant impact on the prevention of problems associated with drug abuse disorder in workplace communities for various reasons. One of them is that a growing number of employers demand the creation of a drug-free work environment, especially when the applicants are about to secure a job and when an employee is suspected of illegal drug use because of a change in behavior or after an unusual accident. Therefore, WDT is a necessary step toward achieving the goal of a drug-free work environment ( Dasgupta et al., 2004 ). Many companies have experienced a drop in productivity associated with the absenteeism of their workers because of the use of illicit drugs. Although WDT can help keep the workplace free of drugs, it remains controversial whether it can always provide accurate results about the status of the applicants. One of these uncertainties is the time of drug testing. If the applicants know the time of testing, they may use adulteration products to yield a negative WDT result. The selection of specimens can be challenging, with urine samples being the gold-standard specimen in WDT; however, in some cases, the applicants cannot provide such samples because of a so-called shy bladder. Another issue is that some drugs can interfere with immunoassay reagents, which increases the workload of WDT. In some applications, urine analysis using confirmation techniques, i.e., chromatography systems coupled with mass spectrometry (MS), is offered, but this also would increase the time, labor, and cost of the analysis. Nevertheless, the way WDT deals with emerging new psychoactive substances (NPS) using primary and confirmation techniques remains the most challenging issue ( Fu et al., 2019 , Salomone et al., 2020 ).
1.1. History of WDT
Fig. 1 provides a historical overview of WDT since the time when President Nixon launched a war on drugs in 1971 and ordered the military to test its service members for drug abuse, especially heroin. This led to the establishment of the first urine drug testing laboratory and the development of forensic drug testing standards and procedures ( Gannon, 2014 ). In 1986, WDT was implemented when President Ronald Reagan gave the executive order to develop a method for keeping the workplace free of drug abuse Bush, 2008 , Willette et al., 1988 . At that time, the establishment of urine drug testing laboratories was needed. Therefore, many institutes were created to design quality assurance programs for drug testing and provide suitable models that must be met by all laboratories performing WDT. The Substance Abuse and Mental Health Administration (SAMHSA) has designed a suitable standard for performing WDT ( Bush, 2008 , White et al., 2023 , D. of H. and H.S, 2017 , DHHS, 1988 ). This program has covered many aspects of WDT and its consequences, including the nature of the drug of abuse to be screened as well as the preparation of a proper employee education program on drug abuse disorder. This process should be conducted under supervision to achieve a workplace free of drug abuse, followed by the introduction of a special program to help employees who have drug abuse disorder and identify employees who can be classified as illegal drug users. These cases should then be subjected to suitable approaches for drug abuse testing and monitoring ( Bush, 2008 ).
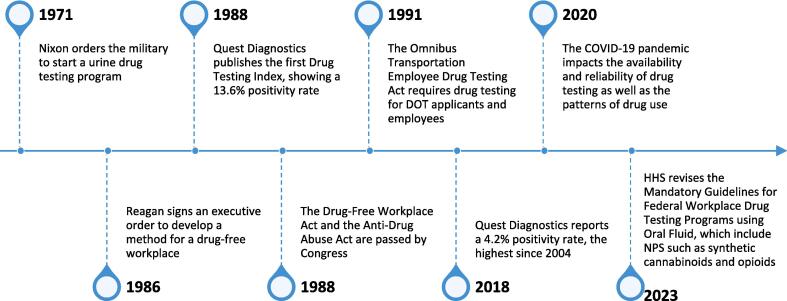
Historical Overview of Workplace Drug Testing.
1.2. WDT structure
The SAMHSA standard and other guidelines prepared by different countries, such as the European Workplace Drug Testing Society ( Taskinen et al., 2017 ), have always considered three stages in the processing of each sample: collection, analysis, and interpretation. First, assessment of sample integrity is a crucial step that is conducted at the site of collection. This involves many essential tests, such as measurement of temperature, color, creatinine level, pH, smell, specific gravity, and nitrate level. Moreover, it is difficult to distinguish a genuine urine sample from an adulterated one ( Kim et al., 2019 , Embers et al., 2019 ; Vikingsson et al., 2022 , Kyle and Kaur, 2020 , Goggin et al., 2017 ). For example, Kim et al. (2019) revealed that the substitution of urine samples with synthetic urine that is currently used in forensic toxicology laboratories for preparing quality control samples can beat WDT, without being able to differentiate gunning from synthetic samples. Consequently, direct supervision and assessment of sample integrity, especially during illicit drug testing, are becoming increasingly necessary to identify adulterated samples.
The analytical stage consists of two steps in the laboratory: first, a primary screening is performed using immunoassays, followed using chromatography instruments (e.g., gas chromatography coupled with MS [GC–MS]) to confirm whether the samples are positive or negative. At this time, the confirmatory step cannot be performed directly because the chromatography machines are sensitive; thus, the specimens need to be extracted before injection into the chromatography machines. In addition to hydrolysis, derivatization procedures may be needed when using GC–MS ( Wu et al., 1999 , Fu, 2019 ), but not when using liquid chromatography (LC)-MS. In the latter case, the urine sample can be injected directly without any sample pretreatment, or the sample can be diluted and injected, including extremely polar glucuronide metabolites of interest ( Fu, 2019 , Gallardo et al., 2009 ). The selection of the best protocol for analysis remains controversial. Each country considers its own drug of interest to be tested during WDT, as immunoassays have an advantage of easy handling by laboratory personnel, whereas high-resolution MS techniques require an expert to interpret the results. Although the samples can be processed rapidly, the interpretation of the results may take several days, which increases the turnaround time (TAT).
Sample collection and analysis are important to aid in the decision-making process; however, the interpretation of both positive and negative results is comprehensive and crucial for finalizing the outcome of the analysis; for example, determining how to interpret any positive or negative result obtained for suspected adulterated samples ( Liu, 1992 ). WDT is an application of forensic toxicology that depends on the testing of metabolites, rather than that of the parent drugs, as urine is the specimen of choice. Some drugs are prodrugs that are converted into extremely polar metabolites, such as glucuronide or sulfate conjugates, which lead to a decrease in the concentration of the parent drug in some cases to a level below the confirmatory cutoff value. Moreover, such drugs require the use of sufficient hydrolysis or a direct method for the detection of drugs and their metabolites. These two approaches are neither time consuming nor expensive, especially for routine testing of a high number of samples ( Fu, 2016 ). In addition, the interpretation of positive or negative results of WDT can be complicated by similarities between different drugs with comparable chemical structures, especially when some of these drugs are illicit and others are legal drugs. Furthermore, the suspected drugs can be metabolites of legal drugs and vice versa; for example, amphetamine is a prescribed drug in the USA and a metabolite of methamphetamine ( Al-Asmari, 2021 ). In addition, I -methamphetamine is a metabolite of selegiline, which is a drug used for treating Parkinson’s disease ( Shin et al., 2021 ). Because d -methamphetamine is an illicit drug and a highly addictive psychostimulant, special chromatographic separation technique should be performed to differentiate these isomers, which is often not available at all WDT laboratories ( Shin et al., 2021 , West et al., 2013 ). In addition to the challenges of distinguishing drug classes with similar chemical structures and identifying source analytes, especially when two drugs share similar metabolic pathways, unintentional ingestion of NPS or illicit drugs may be encountered, which are known as fake drugs or counterfeit drugs in which NPS act as adulterants ( Liu, 1992 , Salomone et al., 2020 , Salomone and Palamar, 2021 , Oliver et al., 2019 ). In the USA, despite NPS being an ingredient, “Molly” is sold as MDMA ( Moeller et al., 2008 , Palamar et al., 2016 ).
1.3. Adulteration
Urine manipulation is a significant problem in drug testing. It is common in various sectors, including WDT, addiction treatment programs, and forensic investigations. The rate of sample manipulation varies from 2 % to 50 % depending on the context ( The Committee Clinical Toxicology, 2005 ). Despite these challenges, comprehensive testing for manipulation should become a standard to ensure the integrity of the drug testing procedures.
Although the SAMHSA standard has been used for WDT and assessment of sample integrity to fully ensure the accuracy of the drug testing results, the reliability of drug testing has often been questioned ( Cody, 1990 ). In fact, it is easy to manipulate urine samples using various substances, which is termed adulteration, to produce false-negative results. Simple adulteration using products such as soap, detergent, and household cleaner has a long history ( Cody and Valtier, 2001 ). The products that are used for adulteration have become more sophisticated, because they have been deliberately designed to cause interference with the immunoassay system and yield a false-negative result ( Liu, 1992 ). The use of adulteration products has increased sharply because employees who are being tested already know that the result would be positive ( Table 1 ). In these cases, the only conceivable approach to pass WDT is to use adulteration products to produce a false-negative result ( Fu, 2019 ). Another reason is the increasing number of drivers who are under the influence of illicit drugs and wish to pass drug tests ( Wissenbach and Steuer, 2023 , The Committee Clinical Toxicology, 2005 ). In many laboratories, a procedure to detect sample adulteration has not yet been implemented ( Fu, 2019 ). Moreover, adulterants have become available on the market, and methods to adulterate specimens have been described by companies that sell adulterants on their websites; anyone can adulterate a drug test specimen by simply surfing internet to obtain detailed information about adulteration ( The Committee Clinical Toxicology, 2005 ).
Common adulteration methods and their detection during workplace drug testing.
Adulteration can be divided into two types: in vivo adulteration and in vitro adulteration ( Fu, 2019 ). In vivo adulteration refers to the ingestion of adulterants by drinking a liquid or performing actions to excrete or eliminate these drugs or their metabolites from the body or to dilute the concentration of the drug ( Fu, 2019 , Fu, 2016 ). Another possibility is to mask these substances to produce a false-negative result via the immunoassay. For example, in vivo adulteration approaches often alter the pH value and drug concentration in the urine sample through the intake of a large amount of water. Diuretics decrease the drug concentration to skew the primary immunoassay drug screening toward a negative result ( Fu, 2019 , Fu, 2016 , Smith and Bluth, 2016 ). Another study reported that the most complicated method is to dilute a urine sample using diuretics combined with vitamins and creatine because normal creatinine can be stimulated by creatine to pass the creatine test. In addition, positive drugs can be masked by vitamins owing to their color ( The Committee Clinical Toxicology, 2005 ). The effects of adulterants on various screening and confirmation methods used for detecting drugs in urine samples are summarized in Table 2 .
Effect of adulterants on different immunoassay methods for drug detection in urine samples.
The first type of adulteration aims to alter sample integrity. The second type of adulteration is employed to interfere with the immunoassay reagent, thus preventing the reaction between antibodies in the immunoassay reagent and the drug metabolites of the sample, which leads to negative results ( The Committee Clinical Toxicology, 2005 ). Some adulteration techniques have been designed to affect a specific immunoassay reagent; for example, detergents have a strong effect on the Enzyme multiplied immunoassay technique (EMIT) reagent but do not affect radioimmunoassay (RIA) results. The third type of adulteration can affect a particular class of drugs; for example, potassium nitrite can prevent a positive result of cannabinoid metabolites. Moreover, many substances have been used as adulterants, such as alcohols, ammonia, ascorbic acid, blood, bleach, detergent, lemon juice, peroxide, golden seal root, and vinegar ( The Committee Clinical Toxicology, 2005 , Cody, 1990 ).
False-negative results have also become a problem, and researchers have proposed many approaches to address this issue ( Fu, 2019 ). They argued that the best way to overcome this issue is to ensure that WDT yields no false-negative results, which seems challenging. A study claimed that urine and blood samples are suitable candidates for drug testing; however, the collection site must be improved using a collection device to ensure that there is no possibility of specimen manipulation among the suspects who are being tested ( Rajšić et al., 2020 ). In the late 80 s, they presented the Franklin collector as a model for use in this context. This container hampers the adulteration of liquids because it takes 1–2 min for adjusting the temperature. In addition, Warmer stated that some adulterants, such as sodium hypochlorite, can be easily smelled, and solid adulterations are detected by observing a residue in the device ( Warner, 1989 ). Another step is that the person who is responsible for collecting urine samples from job applicants for WDT should ask them to empty their pockets and wash their hands. This individual should also ensure that the job applicants do not have a powder or any substance under their fingernails, and the tap water should be turned off in the restroom to prevent its use for dilution of specimens ( Cody, 1990 ). Warner believes that pre-analysis at the collection site is important. Pre-analysis can reveal whether the sample contains an adulterant when the temperature is < 37℃; this would indicate that the sample has been switched. Finally, pH assessment would help the chemist detect the presence of adulterants ( Warner, 1989 ).
1.4. Alternative samples
Other researchers have presented different views; they have suggested that, instead of urine and blood, alternative samples such as hair, saliva, and oral fluid samples should be used, which may be the best approach to avoid false-negative results ( Salomone et al., 2016 , Gallardo et al., 2009 , Tsanaclis et al., 2012 , Brcak et al., 2018 ). SAMHSA and other authorities have published guidelines for the analysis of oral fluid ( Brcak et al., 2018 , D. of H. and H.S, 2020a ) and hair ( Salomone et al., 2016 , D. of H. and H.S, 2020b ) samples for WDT. These samples can be easily collected, and the analytes of interest can be easily detected because the corresponding analytical techniques have been improved over the years and are extremely sensitive and accurate. Moreover, these specimens are difficult to manipulate ( Inoue and Seta 1992 ), and their method of collection is less invasive than that used for urine and blood ( Huestis et al., 1999 ).
It remains unclear which of the following two approaches ensure the validity of the results: collecting samples under tight scrutiny or using unconventional samples instead of urine and blood. Unconventional samples offer many advantages compared with urine and blood samples; in particular, long-term detection using hair samples and considerably short-term detection using oral and saliva samples. In contrast, these samples have limitations, such as extremely low concentrations that require very sensitive and selective testing (e.g., hair testing). Furthermore, some of these unconventional samples have high drug stability, whereas many drugs and their metabolites cannot be detected in urine and blood few days after drug use ( Inoue and Seta, 1992 , Huestis et al., 1999 ). Adulteration can be detected using simple laboratory tests, such as measurement of temperature, pH, and specific gravity as well as smell detection; however, laboratories should set up a standard procedure to detect adulteration. Therefore, the use of these unconventional specimens is less attractive than that of urine samples. Moreover, stealth adulteration at the collection site is challenging, although it is feasible via many techniques. Stealth adulteration could be detected using a simple specimen-check reagent ( The Committee Clinical Toxicology, 2005 , Cody, 1990 , Inoue and Seta, 1992 ).
Although both suggestions have led to the detection of false-negative results during drug testing, the complexity of the problem remains obvious ( Edwards et al., 1993 ). Nonetheless, using alternative subjects is an attractive idea that can provide greater reliability of drug testing in the context of long-term use and recent drug ingestion. However, several simple tests can detect various adulterations. Unfortunately, many adulterations are difficult to detect ( Fu, 2019 ). The use of unconventional samples as complementary specimens to urine and blood samples is recommended. Finally, we must cooperatively fight against adulteration companies to ensure safety. The different sample types used for WDT, and their advantages and disadvantages are summarized in Table 3 .
Advantages and disadvantages of different sample types for workplace drug testing.
1.5. NPS and WDT
NPS are a diverse group of synthetic compounds that mimic the effects of traditional drugs of abuse, such as stimulants, hallucinogens, opioids, and cannabinoids. NPS pose a challenge for drug testing because they are often not covered by routine methods and can have unpredictable pharmacological and toxicological effects. The application of the current SAMHSA standard and other guidelines for WDT is challenging in forensic toxicology testing, especially with the continuous emergence of NPS ( Fu et al., 2019 ). Until recently, none of these protocols included the detection of NPS, even when researchers attempted to design new immunoassays or confirmatory panels that included these novel substances ( Gerona and French, 2022 , Ayala and Kerrigan, 2023 ). It seems that, just for a limited time (as the map of NPS keeps changing annually), some of the NPS detected for the first time and some of the previously known substances that had disappeared were no longer used, and a new NPS would require time for its study and for the collection and understanding of information regarding its metabolic pathways ( Salomone et al., 2020 , United Nation Office on Drugs and Crime, 2022 , Mardal et al., 2019 ). It is challenging to keep a person updated regarding WDT, especially in most scenarios wherein only a few drugs are included in the WDT panel, which is known as traditional or popular drug of abuse ( Fu et al., 2019 , Fu, 2019 , Awuchi et al., 2023 ). The use of NPS instead of traditional drugs of abuse is favorable for drug abusers, as they provide an opportunity to pass WDT, especially for NPS that are not included in the WDT panel. NPS are easily obtainable by the public via internet, especially by those who are curious to have a new different drug experience ( Fu et al., 2019 ). Notably, even when some cross-reactivity is detected using traditional immunoassay reagents, if the testers do not have prior experience with these NPS, the assay will fail to detect them ( Gerona and French, 2022 , Awuchi et al., 2023 ).
The inadvertent consumption of NPS as an adulterant is a significant concern, particularly for users of drugs such as ecstasy. Forensic studies have shown that these substances contain various NPS, leading to unpredictable side effects. The issue extends beyond ecstasy, with novel synthetic opioids (NSO) often sold as heroin or cocaine ( Salomone et al., 2020 ). This situation is further complicated by recent developments in Afghanistan, where the Taliban has shut down most opium farms. This could impact the availability of heroin in Europe, the USA, and other countries, potentially increasing NSO abuse and related mortality. Therefore, more accessible, and cost-effective methods for NPS detection and analysis are urgently needed ( United Nation Office on Drug and Crime, 2023 ).
Testing for NPS in WDT settings presents significant challenges because of the rapid emergence and vast variety of these substances. Laboratory tests for NPS can only be developed after they are available on the market, leading to a continuous lag in testing capabilities for these substances ( Salomone et al., 2020 , Salomone and Palamar, 2021 ). The complexity of testing is compounded by the variability in the reliability and validity of the test kits. Various immunoassays have been used to detect NPS ( Table 4 ). However, these tests have limitations, such as cross-reactivity leading to false-positive results. There is a need for more accessible and cost-effective methods for NPS detection ( Awuchi et al., 2023 ).
Review of immunoassay techniques for workplace drug testing of new psychoactive substances in biological samples.
During the initial rise of NPS in the market, the manufacturers of immunoassay kits had an opportunity to address these emerging drugs. In this context, Castaneto et al. (2015) evaluated the effectiveness of biochip array technology (BAT) immunoassay in detecting designer piperazines in urine samples. The study analyzed 20,017 urine specimens randomly collected at the workplace and revealed that 78 of 840 presumptive positive specimens (9.3 %) were LC–high-resolution MS (HRMS)-positive, with the majority being positive for 1-(3-chlorophenyl) piperazine. Despite improvements in BAT specificity and efficiency, with optimized cutoff values, the study concluded that a high-throughput screening method is still required for the identification of piperazine and NPS. This suggests that, although immunoassays have been implemented with increased cutoff values, they are not yet the preferred method for such analyses. Therefore, despite their potential in this context, further studies are required for immunoassays to become the method of choice in these analyses.
Chhabra et al. (2021) reported results like those of Castaneto et al. (2015) . This study involved the analysis of urine samples from patients in a large healthcare system to detect synthetic opioids. An initial screening was performed, followed by a comprehensive analysis using high-performance tandem mass spectrometry (HPLC-MS/MS). The findings of this study revealed that 65.3 % of the samples contained at least one synthetic opioid, whereas 26.0 % contained two or more synthetic opioids. Notably, over one-third of the samples that initially tested positive for opiates, but not for fentanyl, were later found to contain synthetic opioids upon confirmatory HPLC-MS/MS analysis. This indicates the limitations of the fentanyl immunoassay in terms of sensitivity or the possibility of the emergence of fentanyl analogs without fentanyl ( Salomone and Palamar, 2021 ).
In the realm of analytical laboratory work, advanced testing methods beyond basic immunoassays are urgently needed for the comprehensive detection of new opioids. This necessitates a beneficial collaboration between healthcare institutions and reference laboratories, ensuring appropriate sample collection and the use of confirmatory methods such as targeted HPLC-MS/MS. Laboratories stand to gain from recent advancements in analytical instrumentation and methodologies, which can significantly improve opioid screening approaches. To achieve more reliable toxicosurveillance information, increased investments in laboratory resources are essential ( Salomone and Palamar, 2021 ).
One of the solutions to this problem is using ultra high performance liquid chromatography tandem mass spectrometry (UHPLC-MS/MS) for WDT to cover a large number of traditional drugs and NPS concomitantly (targeted analysis, Table 5 ) ( Maurer, 2021 ). Although this approach is promising, it is time consuming, and its power of identification is low due to the use of low-resolution MS (LRMS), i.e., triple quadrupole MS/MS.
Review of different techniques for workplace drug testing of new psychoactive substances in biological samples using a targeted mass spectrometry approach.
Al-Asmari (2020) investigated the use of LC-MS/MS, specifically the LRMS triple quadrupole variant, in postmortem toxicology investigations. The author not only acknowledged the accuracy of these mass analyzer instruments but also highlighted the challenges associated with initial drug screening using immunoassay techniques, such as high long-term costs and the potential for false-negative results. Al-Asmari discussed the application of LC-MS/MS and GC–MS in routine postmortem analysis, which requires separate analytical methods for different drug classes and metabolites, leading to increased TAT and cost. The author stressed the importance of a method with a wide linear dynamic range (LDR) for accurately measuring analytes at both extremely low and extremely high concentrations. The lethal effect of low concentrations of benzodiazepine when combined with buprenorphine as well as the therapeutic blood levels of trazodone, which can reach up to 2.5 mg/L. Al-Asmari concluded that a wide LDR is essential for the accurate detection and measurement of multiple analytes in various scenarios. This requires appropriate calibration models to avoid the saturation of the MS/MS detector.
In contrast to postmortem toxicology, as detailed by Al-Asmari (2020) , which generally detects any trace levels of drugs and their metabolites across various matrices, WDT operates based on a cutoff value and is typically confined to specific matrices, primarily urine. In 2016, the United Nations Office on Drugs and Crime (UNODC) reported that opioid misuse accounted for 76 % of drug-related fatalities (United Nation Office on Drugs and Crime, 2018) . Furthermore, the UNODC noted unprecedented levels of opium and cocaine production, with methamphetamine and cocaine distribution reaching new areas. Furthermore, the emergence of NPS experienced a fatal and steep increase over the past decade. This indicates that although the misuse of traditional drugs of abuse remains steady and continues to be a leading cause of death, surveillance of NPS should be concurrently performed, thus posing a significant challenge for contemporary WDT.
Salomone et al. (2020) reported that the rise of NPS as a global concern is undeniable, with their usage reported in over 100 countries. The challenges of this situation are manifold, ranging from legislative issues to the limited number of laboratories that can screen and confirm the presence of NPS. These services are vital in scenarios such as WDT and roadside control. However, the deployment of these analytical methods is inconsistent and mostly restricted to specialized laboratories, primarily because of the high costs associated with these advanced analyses. Consequently, although the necessity for NPS detection is being increasingly acknowledged, its implementation is limited by significant hurdles, making it a significant challenge. This predicament highlights the pressing need for more accessible and cost-effective methods for NPS detection and analysis.
In contrast, nontargeted HRMS technology is currently available in most forensic laboratories ( Table 6 ). This technology offers a high-throughput and accurate identification of both known and unknown substances. It is ideally considered capable of replacing immunoassays and providing confirmation using a nontargeted approach based on LC-HRMS techniques ( Salomone et al., 2020 , Fu et al., 2019 ). However, in practice, HRMS in WDT is less preferred than immunoassays because of a longer total TAT for each case. Immunoassays offer a rapid processing of high-load samples, which cannot yet be obtained using HRMS. The most challenging aspect of this analysis is the interpretation of the results. HRMS can be used for screening, but its advantages are limited by the following constraints:
Lack of reference standards and spectral libraries for all NPS
Complexity and variability of data acquisition and processing parameters
Need for advanced data interpretation and validation tools.
The absence of harmonized protocols and quality requirements
The requirement for sufficient resources and trained personnel
Legal and ethical implications of reporting unknown or unregulated substances ( Mardal et al., 2019 ).
Review of different techniques for workplace drug testing of new psychoactive substances in biological samples using a nontargeted mass spectrometry approach.
Malm et al. (2021) investigated how to perform semiquantitative nontargeted screening (NTS) using LC/electrospray ionization (ESI)/HRMS, which is a technique used to identify and estimate the concentrations of unknown compounds in complex samples. They described different strategies for semiquantification, such as the use of surrogate standards, internal standards, calibration curves, response factors, or machine learning models, and discussed their advantages and disadvantages. They also determined the factors affecting the signal intensity and accuracy of semiquantification, such as sample preparation, chromatographic separation, ionization mode, matrix effects, and data quality, and provided recommendations on how to optimize them. Moreover, they provided examples of retrospective analyses, which include re-analysis of the data for new compounds or hypotheses after the initial screening. Additionally, the authors provided a checklist for conducting semiquantitative NTS. The study by Malm et al. is significant because it contributes to the advancement of the knowledge and understanding of NTS as a robust and versatile analytical technique that can reveal novel information about complex samples. However, they also acknowledged the limitations and challenges of performing semiquantitative NTS using LC/ESI/HRMS. The authors revealed that their approach does not directly describe how to determine the cutoff value and positive or negative results in NTS without reference standards. Instead, they reported that the determination depends on the purpose and context of the analysis as well as quality and reliability of the data. They suggested that further research is warranted to validate their results by using other methods or acquiring analytical standards.
Gerona and French (2022) concurred with the abovementioned conclusions and findings, indicating that the rapid emergence and evolution of NPS in the past decade have posed significant challenges to drug testing in clinical laboratories. Some of the unique analytical requirements include the need for comprehensive coverage of various NPS, discovery of unreported NPS through nontargeted data acquisition, and necessity for swift method updates to match the pace of NPS evolution. The constant change in the molecular identities of NPS has complicated both screening and confirmatory assays, with many NPS failing to cross-react with common drug immunoassays, leading to false-negative results and rendering the targeted methods inadequate. The synthetic cannabinoid epidemic in the USA (from 2013 to 2017) exemplified these issues, with new drugs being released more rapidly than those obtained after the validation and implementation of testing methods. However, the advent of HRMS over the past decade offers a promising solution to these analytical challenges. Gerona and French (2022) determined that the combination of LC with HRMS is preferred for analyzing NPS. This is attributed to its capability to process polar and soluble substances without the need for volatility or stability at high temperatures. However, despite these advantages, the adoption of HRMS methods is expensive and requires specialized knowledge. Consequently, its use is currently confined to major reference laboratories and a handful of clinical laboratories affiliated with academic institutions.
Table 6 lists the various applications of nontargeted LC-MS and GC–MS analyses for the detection of NPS in WDT. Nontargeted analyses can overcome these limitations by using HRMS to screen for unknown or unexpected compounds in biological samples, such as urine, blood, hair, or oral fluid. Moreover, nontargeted analysis can be performed using different approaches, such as data-dependent acquisition, data-independent acquisition, post-targeted screening, or retrospective suspect screening. These approaches generate large amounts of data that can be processed using various software tools, such as databases (HighResNPS.com, for example, machine learning, and molecular networking, to identify and prioritize the detected compounds. Furthermore, nontargeted analyses can provide quantitative results for some compounds using calibration curves, response factors, and random forest regression. As shown in Table 6 , nontargeted LC-MS analyses can detect various NPS, including synthetic cannabinoids, stimulants, hallucinogens, benzodiazepines, and other compounds, as well as their metabolites in different matrices and scenarios. Moreover, nontargeted analyses can be used to compare the performance of different sample preparation and extraction methods, such as solid-phase extraction, salting-out liquid–liquid extraction, turbulent flow chromatography, dilute-and-shoot, and urine precipitation. Furthermore, nontargeted analyses can assess the utility and accuracy of the methods in real-world applications, such as examination of patients admitted to emergency departments, wastewater analysis, and metabolomics.
2. Conclusions
WDT remains the best approach for preventing drug abuse in the workplace, despite the challenges posed by NPS and the limitations of the screening methods. The general conclusion is that nontargeted LC-MS and GC–MS analyses are robust and versatile techniques that can enhance the detection and identification of NPS in WDT. Nontargeted analyses can provide new insights into the diversity and dynamics of the NPS market as well as the pharmacology and toxicity of the compounds. Nontargeted analyses can also provide reliable and accurate results for the confirmation and quantification of the compounds. Finally, nontargeted analyses can be easily adapted and expanded to include new compounds and matrices after their emergence.
This research did not receive any specific grant from funding agencies in the public, commercial, or not-for-profit sectors.
CRediT authorship contribution statement
Ahmed Ibrahim Al-Asmari: Conceptualization, Data curation, Formal analysis, Funding acquisition, Investigation, Methodology, Project administration, Resources, Supervision, Validation, Writing – original draft, Writing – review & editing.
Declaration of competing interest
The authors declare that they have no known competing financial interests or personal relationships that could have appeared to influence the work reported in this paper.
Acknowledgements
I would like to express my gratitude to all those who supported me during this research.
- Al-Asmari A.I. Method for the identification and quantification of sixty drugs and their metabolites in postmortem whole blood using liquid chromatography tandem mass spectrometry. Forensic Sci. Int. 2020;309 doi: 10.1016/j.forsciint.2020.110193. [ DOI ] [ PubMed ] [ Google Scholar ]
- Al-Asmari A.I. Methamphetamine-related postmortem cases in Jeddah. Saudi Arabia. Forensic Sci. Int. 2021;321 doi: 10.1016/j.forsciint.2021.110746. [ DOI ] [ PubMed ] [ Google Scholar ]
- Aldubayyan A.A., Castrignanò E., Elliott S., Abbate V. A quantitative LC–MS/MS method for the detection of 16 synthetic cathinones and 10 metabolites and its application to suspicious clinical and forensic urine samples. Pharmaceuticals (basel) 2022;15 doi: 10.3390/ph15050510. [ DOI ] [ PMC free article ] [ PubMed ] [ Google Scholar ]
- Alsenedi K.A., Morrison C. Determination of amphetamine-type stimulants (ATSs) and synthetic cathinones in urine using solid phase micro-extraction fibre tips and gas chromatography-mass spectrometry. Anal. Methods. 2018;10:1431–1440. doi: 10.1039/C8AY00041G. [ DOI ] [ Google Scholar ]
- Alwaeel M., Gomaa R., Ansari N., Nader L. A comparative analysis of three different immunoassay techniques for screening of drugs of abuse in urine and their confirmation using GC-MS & HPLC-MS. J. Forensic Sci. Appl. Toxicol. 2022;22:59–74. doi: 10.21608/ejfsat.2022.96815.1224. [ DOI ] [ Google Scholar ]
- Awuchi C.G., Aja M.P., Mitaki N.B., Morya S., Amagwula I.O., Echeta C.K., Igwe V.S. New psychoactive substances: major groups, laboratory testing challenges, public health concerns, and community-based solutions. J. Chem. 2023;2023:1–36. doi: 10.1155/2023/5852315. [ DOI ] [ Google Scholar ]
- Ayala J., Kerrigan S. Comprehensive toxicological screening of common drugs of abuse, new psychoactive substances and cannabinoids in blood using supported liquid extraction and liquid chromatography–quadrupole time-of-flight mass spectrometry. J. Anal. Toxicol. 2023;47:656–667. doi: 10.1093/jat/bkad069. [ DOI ] [ PubMed ] [ Google Scholar ]
- Barnes A.J., Young S., Spinelli E., Martin T.M., Klette K.L., Huestis M.A. Evaluation of a homogenous enzyme immunoassay for the detection of synthetic cannabinoids in urine. Forensic Sci. Int. 2014;241:27–34. doi: 10.1016/j.forsciint.2014.04.020. [ DOI ] [ PMC free article ] [ PubMed ] [ Google Scholar ]
- Begeman A., Franssen E.J.F. Lack of detection of new amphetamine-like drugs using conventional urinary immunoassays. Ther. Drug Monit. 2018;40:135–139. doi: 10.1097/FTD.0000000000000475. [ DOI ] [ PubMed ] [ Google Scholar ]
- Bengel, C., 2019. D.J. Cooper fails FIBA drug test for using pregnant girlfriend’s urine, reportedly suspended for two years. Available online at https://www.cbssports.com/college-basketball/news/d-j-cooper-fails-fiba-drug-test-for-using-pregnant-girlfriends-urine-reportedly-suspended-for-two-years/ (Viewed on December 15, 2023).
- Brcak M., Beck O., Bosch T., Carmichael D., Fucci N., George C., Piper M., Salomone A., Schielen W., Steinmeyer S., Taskinen S., Weinmann W. European guidelines for workplace drug testing in oral fluid. Drug Test. Anal. 2018;10:402–415. doi: 10.1002/dta.2229. [ DOI ] [ PubMed ] [ Google Scholar ]
- Bronner W., Nyman P., von Minden D. Detectability of phencyclidine and 11-nor-Δ9-tetrahydrocannabinol-9-carboxylic acid in adulterated urine by radioimmunoassay and fluorescence polarization immunoassay. J. Anal. Toxicol. 1990;14:368–371. doi: 10.1093/jat/14.6.368. [ DOI ] [ PubMed ] [ Google Scholar ]
- Bush D.M. The U.S. mandatory guidelines for federal workplace drug testing programs: current status and future considerations. Forensic Sci. Int. 2008;174:111–119. doi: 10.1016/j.forsciint.2007.03.008. [ DOI ] [ PubMed ] [ Google Scholar ]
- Castaneto M.S., Desrosiers N.A., Ellefsen K., Anizan S., Martin T.M., Klette K.L., Huestis M.A. Method validation of the biochip array technology for synthetic cannabinoids detection in urine. Bioanalysis. 2014;6:2919–2930. doi: 10.4155/bio.14.150. [ DOI ] [ PubMed ] [ Google Scholar ]
- Castaneto M.S., Scheidweiler K.B., Gandhi A., Wohlfarth A., Klette K.L., Martin T.M., Huestis M.A. Quantitative urine confirmatory testing for synthetic cannabinoids in randomly collected urine specimens. Drug Test. Anal. 2015;7:483–493. doi: 10.1002/dta.1709. [ DOI ] [ PMC free article ] [ PubMed ] [ Google Scholar ]
- Castaneto M.S., Barnes A.J., Concheiro M., Klette K.L., Martin T.A., Huestis M.A. Biochip array technology immunoassay performance and quantitative confirmation of designer piperazines for urine workplace drug testing. Anal. Bioanal. Chem. 2015;407:4639–4648. doi: 10.1007/s00216-015-8660-z. [ DOI ] [ PubMed ] [ Google Scholar ]
- Castaneto M.S., Huang C., Capps D., Ke P., VanZile M., Calero E. Evaluation of a highly efficient multidrug biochip array technology for a simultaneous and high-throughput urine drug screening in clinical and toxicological settings. Ther. Drug Monit. 2022;44:683–695. doi: 10.1097/FTD.0000000000000981. [ DOI ] [ PubMed ] [ Google Scholar ]
- Chhabra N., Rizvanolli L., Rasin A., Marsden G., Hinami K., Aks S.E. A cross-sectional analysis of fentanyl analog exposures among living patients. Am. J. Drug Alcohol Abuse. 2021;47:344–349. doi: 10.1080/00952990.2021.1891420. [ DOI ] [ PubMed ] [ Google Scholar ]
- Chou, S.-L., Giang, Y.-S., 2007. Elucidation of the US Urine Specimen Validity Testing (SVT) Policies and Performance Evaluation of Five Clinical Parameters for Pre-screening Adulterants in Taiwan’s Opiates Urinalysis.
- Cody J.T., Valtier S. Effects of StealthTM adulterant on immunoassay testing for drugs of abuse. J. Anal. Toxicol. 2001;25:466–470. doi: 10.1093/jat/25.6.466. [ DOI ] [ PubMed ] [ Google Scholar ]
- Cody J.T. Specimen adulteration in drug urinalysis. Forensic Sci. Rev. 1990;2:63–75. [ PubMed ] [ Google Scholar ]
- D. of H. and H.S, 2017. Substance Abuse and Mental Health Services Administration, mandatory guidelines for federal workplace drug testing programs using urine. Vol. 82 FR 7920, effective October 1, 2017. https://www.federalregister.gov/ documents/2017/01/23/2017-00979/mandatory-guidelines-for-federalworkplace-drug-testing-programs (accessed 30 April 2023).
- D. of H. and H.S, 2020a. Substance Abuse and Mental Health Services Administration, Department Of Health And Human Services mandatory guidelines for federal workplace drug testing programs using oral fluid. Vol. 84 FR 57554, effective January 01, 2020. https://www.federalregister.gov/ documents/2019/10/25/2019-22684/mandatory-guidelines-for-federalworkplace-drug-testing-programs-oralfluid (accessed 30 April 2023).
- D. of H. and H.S, 2020b. Substance Abuse and Mental Health Services Administration, Department of Health And Human Services Proposed mandatory guidelines for federal workplace drug testing programs using hair. Vol. 85 FR 56108 Published 9/10/2020. https://www.govinfo.gov/content/pkg/ FR-2020-09-10/pdf/2020-16432.pdf (aceesed 30 April 2023).
- Dasgupta A., Chughtai O., Hannah C., Davis B., Wells A. Comparison of spot tests with AdultaCheck 6 and intect 7 urine test strips for detecting the presence of adulterants in urine specimens. Clin. Chim. Acta. 2004;348:19–25. doi: 10.1016/j.cccn.2004.01.003. [ DOI ] [ PubMed ] [ Google Scholar ]
- De Jager A.D., Warner J.V., Henman M., Ferguson W., Hall A. LC-MS/MS method for the quantitation of metabolites of eight commonly-used synthetic cannabinoids in human urine - an australian perspective. J. Chromatogr. B Analyt. Technol. Biomed. Life Sci. 2012;897:22–31. doi: 10.1016/j.jchromb.2012.04.002. [ DOI ] [ PubMed ] [ Google Scholar ]
- Deville M., Bailly R., Gauthier N., Pitti P., Wachtelaer A., Charlier C. Biochip array technology for new psychoactive substances detection in biological samples: evaluation of the specificity of the randox evidence investigator®. Ann. Clin. Biochem. 2022;59:357–362. doi: 10.1177/00045632221111751. [ DOI ] [ PubMed ] [ Google Scholar ]
- DHHS, 1988. Mandatory guidelines for federal workplace drug testing programs. Fed. Regist. [ DOI ] [ PubMed ]
- Dresen S., Ferreirós N., Gnann H., Zimmermann R., Weinmann W. Detection and identification of 700 drugs by multi-target screening with a 3200 Q TRAP® LC-MS/MS system and library searching. In Anal. Bioanal. Chem. 2010;396:2425–2434. doi: 10.1007/s00216-010-3485-2. [ DOI ] [ PubMed ] [ Google Scholar ]
- Edwards C., Fyfe M.J., Liu R.H., Walia A.S. Evaluation of common urine specimen adulteration indicators. J. Anal. Toxicol. 1993;17:251–252. doi: 10.1093/jat/17.4.251. [ DOI ] [ PubMed ] [ Google Scholar ]
- Ellefsen K.N., Anizan S., Castaneto M.S., Desrosiers N.A., Martin T.M., Klette K.L., Huestis M.A. Validation of the only commercially available immunoassay for synthetic cathinones in urine: randox drugs of abuse V biochip array technology. Drug Testing Anal. 2014;6:728–738. doi: 10.1002/dta.1633. [ DOI ] [ PMC free article ] [ PubMed ] [ Google Scholar ]
- Els C., Jackson T.D., Milen M.T., Kunyk D., Wyatt G., Sowah D., Hagtvedt R., Deibert D., Straube S. Random drug and alcohol testing for preventing injury in workers. Cochrane Database Syst. Rev. 2020;12:CD012921. doi: 10.1002/14651858.CD012921.pub2. [ DOI ] [ PMC free article ] [ PubMed ] [ Google Scholar ]
- Embers D.M., Ravishankar D.A., Din A., Sethi R. Synthetic urines in a buprenorphine clinic. Kans. J. Med. 2019;12:146–147. doi: 10.17161/kjm.v12i4.13263. [ DOI ] [ PMC free article ] [ PubMed ] [ Google Scholar ]
- Fan S.Y., Zang C.Z., Shih P.H., Ko Y.C., Hsu Y.H., Lin M.C., Tseng S.H., Wang D.Y. A LC-MS/MS method for determination of 73 synthetic cathinones and related metabolites in urine. Forensic Sci. Int. 2020;315 doi: 10.1016/j.forsciint.2020.110429. [ DOI ] [ PubMed ] [ Google Scholar ]
- Franz S., Skopp G., Musshoff F. The effect of creatine ingestion on urinary creatinine concentration: does supplementation mask a heavy dilution? Drug Test Anal. 2022;14:162–168. doi: 10.1002/dta.3165. [ DOI ] [ PubMed ] [ Google Scholar ]
- Fu, S., Stove, C., Elliott, S. [Editorial], 2019. Editorial: Advances in analytical methods for drugs of abuse testing. Front. Chem., 7, 589. . [ DOI ] [ PMC free article ] [ PubMed ]
- Fu S. Adulterants in urine drug testing. In Adv. Clin. Chem., Academic Press Inc Cambridge, United States of America. 2016;76:123–163. doi: 10.1016/bs.acc.2016.05.003. [ DOI ] [ PubMed ] [ Google Scholar ]
- Fu, S., 2019. How do people try to beat drugs test? Effects of synthetic urine, substituted urine, diluted urine, and in vitro urinary adulterants on drugs of abuse testing. In Crit. Issues Alcohol. Drugs Abuse Test. .
- Gallardo E., Barroso M., Queiroz J.A. LC-MS: a powerful tool in workplace drug testing. Drug Test. Anal. 2009;1:109–115. doi: 10.1002/dta.26. [ DOI ] [ PubMed ] [ Google Scholar ]
- Gannon, L., 2014. Ask the experts: the history of drug testing, Vols. 1–1. https://blog.employersolutions.com/ask-the-experts-the-history-of-drug-testing (accessed 15 December 2023).
- Gerace E., Caneparo D., Borio F., Salomone A., Vincenti M. Determination of several synthetic cathinones and an amphetamine-like compound in urine by gas chromatography with mass spectrometry. method validation and application to real cases. J. Sep. Sci. 2019;42:1577–1584. doi: 10.1002/jssc.201801249. [ DOI ] [ PubMed ] [ Google Scholar ]
- Gerona R.R., French D. Drug testing in the era of new psychoactive substances. In Adv. Clin. Chem. 2022;111:217–263. doi: 10.1016/bs.acc.2022.08.001. [ DOI ] [ PubMed ] [ Google Scholar ]
- Goggin M.M., Tann C.M., Miller A., Nguyen A., Janis G.C. Catching fakes: new markers of urine sample validity and invalidity. J. Anal. Toxicol. 2017;41:121–126. doi: 10.1093/jat/bkw119. [ DOI ] [ PubMed ] [ Google Scholar ]
- Gundersen P.O.M., Spigset O., Josefsson M. Screening, quantification, and confirmation of synthetic cannabinoid metabolites in urine by UHPLC–QTOF–MS. Drug Test. Anal. 2019;11:51–67. doi: 10.1002/dta.2464. [ DOI ] [ PMC free article ] [ PubMed ] [ Google Scholar ]
- Helander A., Stojanovic K., Villén T., Beck O. Detectability of fentanyl and designer fentanyls in urine by 3 commercial fentanyl immunoassays. Drug Test. Anal. 2018;10 doi: 10.1002/dta.2382. [ DOI ] [ PubMed ] [ Google Scholar ]
- Helfer A.G., Michely J.A., Weber A.A., Meyer M.R., Maurer H.H. LC-HR-MS/MS standard urine screening approach: pros and cons of automated on-line extraction by turbulent flow chromatography versus dilute-and-shoot and comparison with established urine precipitation. J Chromatogr. B Analyt. Technol. Biomed. Life Sci. 2017;1043:138–149. doi: 10.1016/j.jchromb.2016.06.036. [ DOI ] [ PubMed ] [ Google Scholar ]
- Hong W.Y., Ko Y.C., Lin M.C., Wang P.Y., Chen Y.P., Chiueh L.C., Shih D.Y.C., Chou H.K., Cheng H.F. Determination of synthetic cathinones in urine using gas chromatography-mass spectrometry techniques. J. Anal. Toxicol. 2016;40:12–16. doi: 10.1093/jat/bkv108. [ DOI ] [ PubMed ] [ Google Scholar ]
- Huestis M.A., Oyler J.M., Cone E.J., Wstadik A.T., Schoendorfer D., Joseph R.E. Sweat testing for cocaine, codeine and metabolites by gas chromatography-mass spectrometry. J. Chromatogr. B Biomed. Sci. Appl. 1999;733:247–264. doi: 10.1016/S0378-4347(99)00246-7. [ DOI ] [ PubMed ] [ Google Scholar ]
- Huppertz L.M., Kneisel S., Auwärter V., Kempf J. A comprehensive library-based, automated screening procedure for 46 synthetic cannabinoids in serum employing liquid chromatography-quadrupole ion trap mass spectrometry with high-temperature electrospray ionization. J. Mass Spectrom. 2014;49:117–127. doi: 10.1002/jms.3328. [ DOI ] [ PubMed ] [ Google Scholar ]
- Inoue T.S.S., Seta S. Analysis of drugs in unconventional samples. Forensic Sci. Rev. 1992;4:89–107. [ PubMed ] [ Google Scholar ]
- Kim V.J., Okano C.K., Osborne C.R., Frank D.M., Meana C.T., Castaneto M.S. , Can synthetic urine replace authentic urine to “beat” workplace drug testing? Drug Test Anal. 2019;11:331–335. doi: 10.1002/dta.2497. [ DOI ] [ PubMed ] [ Google Scholar ]
- Kyle P.B., Kaur J. Evaluating novel markers for specimen validity testing. Arch. Pathol. Lab. Med. 2020;144:168–171. doi: 10.5858/arpa.2019-0197-OA. [ DOI ] [ PubMed ] [ Google Scholar ]
- Liu R.H., Wu C.H., Chen Y.J., Chang C.D., Linville J.G., Canfield D.V. Intensity of the internal standard response as the basis for reporting a test specimen as negative or inconclusive. J. Anal. Toxicol. 2007;31:549–554. doi: 10.1093/jat/31.9.549. [ DOI ] [ PubMed ] [ Google Scholar ]
- Liu R.H. Important considerations in the interpretation of forensic urine drug test results. Forensic Sci. Rev. 1992;4:51–65. [ PubMed ] [ Google Scholar ]
- Lung D., Wilson N., Chatenet F.T., Lacroix C., Gerona R. Non-targeted screening for novel psychoactive substances among agitated emergency department patients. Clin. Toxicol. (Phila) 2016;54:319–323. doi: 10.3109/15563650.2016.1139714. [ DOI ] [ PubMed ] [ Google Scholar ]
- Luong S., Ung A.T., Kalman J., Fu S. Transformation of codeine and codeine-6-glucuronide to opioid analogues by urine adulteration with pyridinium chlorochromate: potential issue for urine drug testing. Rapid Commun. Mass Spectrom. 2014;28:1609–1620. doi: 10.1002/rcm.6935. [ DOI ] [ PubMed ] [ Google Scholar ]
- Malm L., Palm E., Souihi A., Plassmann M., Liigand J., Kruve A. Guide to semi-quantitative non-targeted screening using lc/esi/hrms. Molecules. 2021;26 doi: 10.3390/molecules26123524. [ DOI ] [ PMC free article ] [ PubMed ] [ Google Scholar ]
- Mardal M., Andreasen M.F., Mollerup C.B., Stockham P., Telving R., Thomaidis N.S., Diamanti K.S., Linnet K., Dalsgaard P.W. HighResNPS.com: an online crowd-sourced HR-MS database for suspect and non-targeted screening of new psychoactive substances. J. Anal. Toxicol. 2019;43:520–527. doi: 10.1093/jat/bkz030. [ DOI ] [ PubMed ] [ Google Scholar ]
- Matriciani B., Huppertz B., Keller R., Weiskirchen R. False-negative results in the immunoassay analysis of drugs of abuse: can adulterants be detected by sample check test? Ann. Clin. Biochem. 2018;55:348–354. doi: 10.1177/0004563217725089. [ DOI ] [ PubMed ] [ Google Scholar ]
- Maurer H.H. Hyphenated high-resolution mass spectrometry—the “all-in-one” device in analytical toxicology? Anal. Bioanal. Chem. 2021;413:2303–2309. doi: 10.1007/s00216-020-03064-y. [ DOI ] [ PMC free article ] [ PubMed ] [ Google Scholar ]
- Mikkelsen S.L., Ash K.O. Adulterants causing false negatives in illicit drug testing. Clin. Chem. 1988;34:2333–2336. doi: 10.1093/clinchem/34.11.2333. [ DOI ] [ PubMed ] [ Google Scholar ]
- Moeller K.E., Lee K.C., Kissack J.C. Urine drug screening: practical guide for clinicians. Mayo Clin. Proc. 2008;83:66–76. doi: 10.4065/83.1.66. [ DOI ] [ PubMed ] [ Google Scholar ]
- Nieddu M., Trignano C., Burrai L., Pirisi M.A., Boatto G. Cross-reactivities of 41 new amphetamine designer drugs to EMIT®immunoassays. Forensic Toxicol. 2013;31:133–137. doi: 10.1007/s11419-012-0156-3. [ DOI ] [ Google Scholar ]
- Nuclear Regulatory Commission, 2022. Fitness for duty drug testing requirements. https://www.govinfo.gov/content/pkg/FR-2022-11-22/pdf/2022-24903.pdf (accessed 15 December 2023).
- Oliver C.F., Palamar J.J., Salomone A., Simmons S.J., Philogene-Khalid H.L., Stokes-McCloskey N., Rawls S.M. Synthetic cathinone adulteration of illegal drugs. Psychopharmacol. (Berl.) 2019;236:869–879. doi: 10.1007/s00213-018-5066-6. [ DOI ] [ PMC free article ] [ PubMed ] [ Google Scholar ]
- Palamar J.J., Salomone A., Vincenti M., Cleland C.M. Detection of “bath salts” and other novel psychoactive substances in hair samples of ecstasy/MDMA/“Molly” users. Drug Alcohol Depend. 2016;161:200–205. doi: 10.1016/j.drugalcdep.2016.02.001. [ DOI ] [ PMC free article ] [ PubMed ] [ Google Scholar ]
- Partridge E., Trobbiani S., Stockham P., Scott T., Kostakis C. A validated method for the screening of 320 forensically significant compounds in blood by LC/QTOF, with simultaneous quantification of selected compounds. J. Anal. Toxicol. 2018;42:220–231. doi: 10.1093/jat/bkx108. [ DOI ] [ PubMed ] [ Google Scholar ]
- Paul M., Ippisch J., Herrmann C., Guber S., Schultis W. Analysis of new designer drugs and common drugs of abuse in urine by a combined targeted and untargeted LC-HR-QTOFMS approach. Anal. Bioanal. Chem. 2014;406:4425–4441. doi: 10.1007/s00216-014-7825-5. [ DOI ] [ PubMed ] [ Google Scholar ]
- Petrie M., Lynch K.L., Ekins S., Chang J.S., Goetz R.J., Wu A.H.B., Krasowski M.D. Cross-reactivity studies and predictive modeling of “bath salts” and other amphetamine-type stimulants with amphetamine screening immunoassays. Clin. Toxicol. (phila) 2013;51:83–91. doi: 10.3109/15563650.2013.768344. [ DOI ] [ PubMed ] [ Google Scholar ]
- Pham A.Q.N., Kelly T., Fu S. Urine adulteration: can bleach be used to mask MDMA use? Anal Methods. 2013;5:3948–3955. doi: 10.1039/C3AY40543E. [ DOI ] [ Google Scholar ]
- Puzyrenko A., Wang D., Schneider R., Wallace G., Schreiber S., Brandt K., Gunsolus I.L. Urine drug screening in the era of designer benzodiazepines: comparison of three immunoassay platforms, LC-QTOF-MS and LC-MS-MS. J. Anal. Toxicol. 2022;46:712–718. doi: 10.1093/jat/bkab108. [ DOI ] [ PubMed ] [ Google Scholar ]
- Quick Fix Synthetic Urine, 2023b. The best synthetic urine by spectrum labs. https://quickfixurine.com/ (Accessed 15 December 2023).
- Rajšić I., Javorac D., Tatović S., Repić A., Dukic-Cosić D., Đorđević S., Lukić V., Bulat Z. Effect of urine adulterants on commercial drug abuse screening test strip results. Arh. Hig. Rada. Toksikol. 2020;71:87–93. doi: 10.2478/aiht-2020-71-3315. [ DOI ] [ PMC free article ] [ PubMed ] [ Google Scholar ]
- Regester L.E., Chmiel J.D., Holler J.M., Vorce S.P., Levine B., Bosy T.Z. Determination of designer drug cross-reactivity on five commercial immunoassay screening kits. J. Anal. Toxicol. 2015;39:144–151. doi: 10.1093/jat/bku133. [ DOI ] [ PubMed ] [ Google Scholar ]
- Reinstadler V., Lierheimer S., Boettcher M., Oberacher H. A validated workflow for drug detection in oral fluid by non-targeted liquid chromatography-tandem mass spectrometry. Anal. Bioanal. Chem. 2019;411:867–876. doi: 10.1007/s00216-018-1504-x. [ DOI ] [ PMC free article ] [ PubMed ] [ Google Scholar ]
- Rodrigues W.C., Catbagan P., Rana S., Wang G., Moore C. Detection of synthetic cannabinoids in oral fluid using ELISA and LC-MS-MS. J. Anal. Toxicol. 2013;37:526–533. doi: 10.1093/jat/bkt067. [ DOI ] [ PubMed ] [ Google Scholar ]
- Rodriguez-Cruz S.E. Evaluating the sensitivity, stability, and cross-reactivity of commercial fentanyl immunoassay test strips. J. Forensic Sci. 2023;68:1555–1569. doi: 10.1111/1556-4029.15332. [ DOI ] [ PubMed ] [ Google Scholar ]
- Rossi B., Freni F., Vignali C., Stramesi C., Collo G., Carelli C., Moretti M., Galatone D., Morini L. Comparison of two immunoassay screening methods and a lc-ms/ms in detecting traditional and designer benzodiazepines in urine. Molecules. 2021;27 doi: 10.3390/molecules27010112. [ DOI ] [ PMC free article ] [ PubMed ] [ Google Scholar ]
- Salomone A., Palamar J.J. Toxicosurveillance of novel opioids: just screening tests may not be enough. Am. J. Drug Alcohol Abuse. 2021;47:271–272. doi: 10.1080/00952990.2021.1917588. [ DOI ] [ PMC free article ] [ PubMed ] [ Google Scholar ]
- Salomone A., Tsanaclis L., Agius R., Kintz P., Baumgartner M.R. European guidelines for workplace drug and alcohol testing in hair. Drug Test. Anal. 2016;8:996–1004. doi: 10.1002/dta.1999. [ DOI ] [ PubMed ] [ Google Scholar ]
- Salomone A., Palamar J.J., Vincenti M. Should NPS be included in workplace drug testing? Drug Test Anal. 2020;12:191–194. doi: 10.1002/dta.2749. [ DOI ] [ PMC free article ] [ PubMed ] [ Google Scholar ]
- Scheidweiler K.B., Huestis M.A. Simultaneous quantification of 20 synthetic cannabinoids and 21 metabolites, and semi-quantification of 12 alkyl hydroxy metabolites in human urine by liquid chromatography-tandem mass spectrometry. J. Chromatogr. A. 2014;1327:105–117. doi: 10.1016/j.chroma.2013.12.067. [ DOI ] [ PMC free article ] [ PubMed ] [ Google Scholar ]
- Secaucus, N.J., 2019. Workforce Drug Testing Positivity Climbs to Highest Rate Since 2004, According to New Quest Diagnostics Analysis.
- Shi Y., Liu M., Li X., Xu N., Yuan S., Yu Z., Xiang P., Wu H. Simultaneous screening of 239 synthetic cannabinoids and metabolites in blood and urine samples using liquid chromatography–high resolution mass spectrometry. J. Chromatogr. A. 2022;1663 doi: 10.1016/j.chroma.2021.462743. [ DOI ] [ PubMed ] [ Google Scholar ]
- Shin I., Choi H., Kang S., Kim J., Park Y., Yang W. Detection of l-methamphetamine and l-amphetamine as selegiline metabolites. J. Anal. Toxicol. 2021;45:99–104. doi: 10.1093/jat/bkaa058. [ DOI ] [ PubMed ] [ Google Scholar ]
- Skinnider M.A., Mérette S.A.M., Pasin D., Rogalski J., Foster L.J., Scheuermeyer F., Shapiro A.M. Identification of emerging novel psychoactive substances by retrospective analysis of population-scale mass spectrometry data sets. Anal. Chem. 2023;95:17300–17310. doi: 10.1021/acs.analchem.3c03451. [ DOI ] [ PubMed ] [ Google Scholar ]
- Smith M.P., Bluth M.H. Common interferences in drug testing. Clin. Lab. Med. 2016;36:663–671. doi: 10.1016/j.cll.2016.07.006. [ DOI ] [ PubMed ] [ Google Scholar ]
- Snyder M.L., Jarolim P., Melanson S.E.F. A new automated urine fentanyl immunoassay: technical performance and clinical utility for monitoring fentanyl compliance. Clin. Chim. Acta. 2011;412:946–951. doi: 10.1016/j.cca.2011.01.029. [ DOI ] [ PubMed ] [ Google Scholar ]
- Spinelli, E., Barnes, A.J., Young, S., M.S., 2015 Castaneto, T.M. Drug Test. Anal., K.L. Klette, Martin, M.A. Huestis, Performance characteristics of an ELISA screening assay for urinary synthetic cannabinoids, 7 10.1002/dta.1702. [ DOI ] [ PubMed ]
- Sundström M., Pelander A., Ojanperä I. Comparison of post-targeted and pre-targeted urine drug screening by UHPLC-HR-QTOFMS. J. Anal. Toxicol. 2017;41:623–630. doi: 10.1093/JAT/BKX044. [ DOI ] [ PubMed ] [ Google Scholar ]
- Taskinen S., Beck O., Bosch T., Brcak M., Carmichael D., Fucci N., George C., Piper M., Salomone A., Schielen W., Steinmeyer S., Weinmann W. European guidelines for workplace drug testing in urine. Drug Test. Anal. 2017;9:853–865. doi: 10.1002/dta.2178. [ DOI ] [ PubMed ] [ Google Scholar ]
- The Committee Clinical Toxicology/Drug of Abuse of the International Association for Therapeutic Drug Monitoring and Clinical Toxicology, The Effect of Urine Manipulation on Substances Abuse Testing, Bulletin of the International Association of Forensic Toxicologists XXXV, 2005, 18–24.
- Trobbiani S., Stockham P., Kostakis C. A method for the sensitive targeted screening of synthetic cannabinoids and opioids in whole blood by LC-QTOF-MS with simultaneous suspect screening using HighResNPS.com. NPS.com J. Anal. Toxicol. 2023;47:807–817. doi: 10.1093/jat/bkad063. [ DOI ] [ PubMed ] [ Google Scholar ]
- Tsanaclis L.M., Wicks J.F.C., Chasin A.A.M. Workplace drug testing, different matrices different objectives. Drug Test. Anal. 2012;4:83–88. doi: 10.1002/dta.399. (PubMed: 22362574) [ DOI ] [ PubMed ] [ Google Scholar ]
- United Nation Office on Drug and Crime, 2023a. UNODC early warning advisory on new psychoactive substances. https://www.unodc.org/LSS/Page/NPS (accessed 10 November 2023).
- United Nation Office on Drugs and Crime, 2018. World Drug Report 2018. https://www.unodc.org/wdr2018/prelaunch/WDR18_Booklet_1_EXSUM.pdf . Accessed October 9, 2019.
- United Nation Office on Drugs and Crime , 2022. World Drug Report 2022, Vienna, Austria.
- Vikingsson S., Krauss S.T., Winecker R.E., Flegel R.R., Hayes E.D. Update on urine adulterants and synthetic urine samples to subvert urine drug testing. J. Anal. Toxicol. 2022;46:697–704. doi: 10.1093/jat/bkac029. [ DOI ] [ PubMed ] [ Google Scholar ]
- Wang G., Huynh K., Barhate R., Rodrigues W., Moore C., Coulter C., Vincent M., Soares J. Development of a homogeneous immunoassay for the detection of fentanyl in urine. Forensic Sci. Int. 2011;206:127–131. doi: 10.1016/j.forsciint.2010.07.022. [ DOI ] [ PubMed ] [ Google Scholar ]
- Warner A. Interference of common household chemicals in immunoassay methods for drugs of abuse. Clin. Chem. 1989;35:648–651. doi: 10.1093/clinchem/35.4.648. [ DOI ] [ PubMed ] [ Google Scholar ]
- West R., Pesce A., West C., Mikel C., Velasco J., Gonzales E., Dizon Z., Almazan P., Latyshev S. Differentiating medicinal from illicit use in positive methamphetamine results in a pain population. J. Anal. Toxicol. 2013;37:83–89. doi: 10.1093/jat/bks096. [ DOI ] [ PubMed ] [ Google Scholar ]
- White R.M., Winecker R.E., Drug E.-R. Testing of biological matrices: urine, oral fluid and hair comparison. TIAFT Bull. 2023;53:9–15. [ Google Scholar ]
- Willette, R., Proficiency Testing, Quality Control Program, 1988. In: Hawks, R., Chiang (Eds.), Urine Testing for Drugs of Abuse. NIDA Research Monograph), National Institute on Drug Abuse, pp. 73–120.
- Wissenbach D.K., Steuer A.E. Advances in testing for sample manipulation in clinical and forensic toxicology-Part a: urine samples. Anal. Bioanal. Chem. 2023;415:5101–5115. doi: 10.1007/s00216-023-04711-w. [ DOI ] [ PMC free article ] [ PubMed ] [ Google Scholar ]
- Wu A.H.B., Forte E., Casella G., Sun K., Hemphill G., Foery R., Schanzenbach H. CEDIA for screening drugs of abuse in urine and the effect of adulterants. J. Forensic Sci. 1995;40:614–618. doi: 10.1520/jfs13836j. [ DOI ] [ PubMed ] [ Google Scholar ]
- Wu A.H.B., Bristol B., Sexton K., Cassella-McLane G., Holtman V., Hill D.W. Adulteration of urine by “urine luck”. Clin. Chem. 1999;45:1051–1057. doi: 10.1093/clinchem/45.7.1051. [ DOI ] [ PubMed ] [ Google Scholar ]
- View on publisher site
- PDF (873.4 KB)
- Collections
Similar articles
Cited by other articles, links to ncbi databases.
- Download .nbib .nbib
- Format: AMA APA MLA NLM
Add to Collections
An official website of the United States government
Official websites use .gov A .gov website belongs to an official government organization in the United States.
Secure .gov websites use HTTPS A lock ( Lock Locked padlock icon ) or https:// means you've safely connected to the .gov website. Share sensitive information only on official, secure websites.
- Publications
- Account settings
- Advanced Search
- Journal List

Analytical Methods Used for the Detection and Quantification of Benzodiazepines
Zidane qriouet, zineb qmichou, nadia bouchoutrouch, hassan mahi, yahia cherrah, hassan sefrioui.
- Author information
- Article notes
- Copyright and License information
Academic Editor: Josep Esteve-Romero
Corresponding author.
Received 2019 Apr 17; Accepted 2019 May 26; Collection date 2019.
This is an open access article distributed under the Creative Commons Attribution License, which permits unrestricted use, distribution, and reproduction in any medium, provided the original work is properly cited.
The prescription of psychotropic drugs, especially benzodiazepines (BZDs), occupies a preponderant place in the management of mental illnesses. Indeed, the BZDs have been used in different therapeutic areas including insomnia, anxiety, seizure disorders, or general anesthesia. Unfortunately, these drugs are present in the illegal street market, leading to a lot of drug abuse amongst some addicted users, road insecurity, and suicide. Hence, it has become essential to analyze the BZDs drugs in human biological specimens for drug abuse in forensic sciences. The present review provides a summary of sample preparation techniques (solid-phase extraction and Liquid-liquid phase extraction) and the methods for the detection and quantification of BZDs molecules in the commonly used biological specimens over the ten last years which may potentially lead to better and accurate evaluation of the physiological state of a given person. The commonly used methods for the detection and quantification of BZDs include nuclear magnetic resonance (NMR), chromatography (GC-MS, HPLC, and TLC), immunoassay (ELISA, RIA, LFA, CEDEA, FPIA, and KIMS), and electroanalytical methods (voltammetry and potentiometry).
1. Introduction
Benzodiazepines (BZDs) are a class of psychoactive drugs, introduced in the 1960s, creating a revolution in the field of anxiolytic drugs [ 1 ]. The list of the most prescribed BZDs in the world is categorized, according to their main property, as anticonvulsant, sedative, anxiolytic, amnesic, and hypnotic [ 2 , 3 ]. BZDs were amongst the most prescribed psychotropic drugs in western countries, particularly in France [ 4 ]. Their annual prevalence use is around 2% to 17% [ 4 , 5 ] and varies with countries and from one scientific study to another. The abuse or misuse of BZDs is one of the potential serious social problems worldwide. Their prescription must be reassessed after a short period (12 weeks), because long-term BZDs use has also been described as causing cognitive effects (increasing incidence of dementia), dependence, and withdrawal [ 6 – 8 ]. Indeed, the official international recommendations concerning the use of this therapeutic BZDs molecules are frequently updated, emphasizing short and uninterrupted prescription periods in order to avoid possible abuse of these drugs.
To prevent the BZDs increasing incidence of abuse in the world, the researchers focus on the development of innovative, highly sensitive, and accurate methods to analyze the BZDs and their metabolites. Indeed, the determination of BZDs in biological fluids is essential in clinical assays as well as in forensics and toxicological studies.
The commonly used biological specimens for the analysis of BZDs are blood, urine, and saliva. The major factors evaluated during this analysis are related to the presence or absence of the target BZDs molecules or their related metabolite in the tested samples.
Currently, there are 2 types of BZDs analysis: 1, the screening (qualitative or semiquantitative methods) and 2, the dosage (quantitative methods). However, a toxicological screening usually involves several screening and dosing methods.
Many techniques more and more efficient do exist (GC-MS, HPLC, RMN, etc.). The possibilities of detection and quantification of BZDs will still depend on the methods available in each laboratory. Indeed, the clinician or the scientist must know which ones are accessible to him, their limits of sensitivity and accuracy, and their time constraints in order not to prescribe them unnecessarily. Thus, the method allowing quick, sensitive, and cost-effective detection of BZDs does not exist yet.
Our review covers the analytical methods for BZDs determination and sample preparation techniques used in the studies published over the past ten years.
2. Biological Specimens Commonly Used for the Analysis of BZDs
The detection and quantification of BZDs can be done in different biological matrices including human breast milk [ 9 ], saliva [ 10 – 12 ], blood [ 13 – 15 ], blood serum [ 16 ], plasma [ 9 ], urine [ 14 , 17 – 20 ], nails [ 21 ], hair [ 22 ], meconium [ 23 ], edible animal tissues and feed [ 24 ], and expired air [ 25 ]. However, it is important to keep in mind that whatever the biological matrix used for the screening, the BZDs concentrations depend on many factors, namely, the consumed dose, the quality of the product, the mode of consumption, the metabolism of the consumer, the body weight of the user, and his state of health.
In the present review, we will discuss the most commonly used biological matrices for psychotropic drug analysis [ 26 ]. A special focus will be on saliva, urine, and blood matrices.
2.1. Saliva
Saliva is one of the interesting biological specimens for detecting a recent psychotropic drugs intake compared to urine [ 27 ]. It is considered as one of the major arguments in favor of its use in health, at work or at the roadside by the police in the detection of narcotics used by drivers of vehicles involved in traffic accidents [ 28 , 29 ]. Indeed, saliva was approved as the screening method [ 30 ] in 2011 by the Substance Abuse and Mental Health Services Administration (SAMHSA, USA).
Amongst the drugs detected in human saliva, we have the amphetamines, 3,4-methylenedioxy-methamphetamine (MDMA), cocaine, opiates (heroin and codeine), cannabis, and BZDs [ 10 – 12 , 19 , 28 , 31 – 34 ].
The main benefits of using saliva samples for psychotropic drug screening include its noninvasive nature, ease of sampling, handling does not raise difficulties with the intimacy of the person, and adulteration is very difficult. In fact, only a visual checkup by trained staff is required [ 35 ]. In addition, the salivary screening assay has the advantage of testing for parent molecules rather than metabolites. The pharmacokinetic profile of the molecules appears to be parallel in saliva and plasma, although the ratio of blood and salivary levels is inconsistent for a number of molecules. Hence, these pharmacokinetic characteristics represent a significant advantage when one seeks to establish a formal link between an exposure and the occurrence of an accident. It is not necessarily the same when one seeks to establish the existence of an impregnation and a longer detection time is more informative [ 35 ].
However, the saliva collecting method is very crucial and can influence the results. The contamination of the saliva sample by other substances such as certain foods, condiments, drinks, or bacteria cannot be formally excluded [ 36 ]. Also, there is no standard method for treating and collecting samples before analysis, and the choice of sampling device and the treatment of the saliva have been approached very differently by different authors [ 11 , 12 , 37 ].
Urine drug tests are the most common types of tests used among medical professionals. Many countries adopted these tests for road control, and many jobs now require a screening in order to apply. In research area, many studies used urine as biological matrix for drug analysis [ 14 , 17 – 20 , 38 ]. Indeed, the search for illicit drugs in the urine provides information on chronic or recent consumption. Yet, urine-screening tests are more difficult to implement, because the sampling presents major constraints. Urine tests are more common because they are noninvasive, are fast, offer the advantage of providing a large sample volume, and are able to qualitatively detect a wide range of substances including BZD. These tests offer also a lengthy amount of detection time; thus, the detection of drugs depends on the frequency and quantity of drugs used (1 to 4 days for most drugs), the development and validation of more sensitive and accurate analysis methods, the cutoff levels, the standard recognized protocols, and the standards of practice.
However, the urine needs to be properly stored to provide stable and valid results, and due to the privacy of providing samples, it can be altered before the analysis. To respect the person's privacy and also to avoid adulteration of the levy, it is necessary to impose adapted premises, trained personnel, examination of the color, density, measurement of the pH, and temperature of the sample immediately after it was carried out.
Blood is probably the only medium with the potential to indicate whether an individual is under the influence of BZD, or not, at the time of collection. It is considered as an essential element in the control of drug abuse in the workplace.
From a practical point of view, blood sample is the most restrictive to collect, compared to saliva or even urine. Indeed, it needs to be performed by qualified medical personnel in a laboratory. However, this process takes time, and sometimes, it can mean the difference in accuracy between a positive or negative test.
Blood tests can be performed to quantify the levels of certain BZDs and their metabolites but are more rarely practiced because of their invasive procedure.
Blood unlike urine has the advantage of being impossible to be impaired, and moreover, there is a proven relationship between the amount absorbed and the blood level and therefore effects on the central nervous system (dose/concentration relationship and dose/effect) [ 39 ]. The detection window in the blood is narrower than urine, and the concentrations are lower. Therefore, a sensitive and a very specific confirmation technique is mandatory for the detection of BZDs and their metabolites in the blood like HPLC or LC-MS/MS [ 11 , 17 – 19 ], gas chromatography, or GC-Mass spectrometry [ 10 , 13 – 15 , 18 ].
These matrices contain a multitude of substances endogenous (proteins in the blood or fatty acids in urine) in amounts well above those compounds and their metabolites to be quantified. Many endogenous compounds have reactive functional groups (such as the carboxylic functions of amino acids or fatty acids) that can participate in derivatization reactions and interfere with the analysis of the compounds of interest. Preprocessing of samples is therefore a fundamental step for this type of analysis. In this regard, modern isolation techniques, e.g., solid-phase extraction, are necessary [ 13 ].
3. Sample Preparation (Pretreatment and Extraction)
Pretreatment of the sample aims to isolate and concentrate the xenobiotic ( s ) in a matrix while extracting as little as possible of the endogenous compounds prior to analysis. This is most certainly the most important step in the analytic process. The diversity of biological samples, entrusted to toxicological laboratories for analysis, show complex matrices (blood, urine, hair, saliva, meconium, etc.). From this observation, it is well known why good preparation has a direct influence on the limit of detection, repeatability, and reproducibility of the analysis. Over the years, various procedures have been developed including liquid-liquid extraction (LLE), solid-phase extraction (SPE), molecularly imprinted solid-phase extraction (MISPE), microextraction (SPME and LPME), supercritical fluid extraction (SFE), online solid-phase extraction, ASPEC (automated sample preparation with extraction columns) system, column switching, dispersive liquid-liquid microextraction (DLLME), nonextraction procedures (dialysis of biofluids using a semipermeable gold membrane direct injection of crude samples after protein precipitation), and fabric phase sorptive extraction (FPSE) that combines the extraction of SPME/SPE mode into a single technology platform. In this review, we will describe the LLE and the SPE methods.
3.1. Solid-Phase Extraction (SPE)
Solid-phase extraction is based on the sharing of compounds between a liquid phase, the sample, and a stationary phase, the adsorbent. It usually consists of four stages.
The first step is the conditioning of the stationary phase. It allows it to be wetted by means of an organic solvent and to activate the sites of retention, the seat of the molecular interactions. A hydrophobic support is conditioned by an organic solvent (most often methanol) and then by a solvent whose ionic and pH characteristics are as close as possible to the solvent of the sample (generally water). The second step is the deposit of the sample. The goal is to cause a quantitative retention of analytes of interest on the stationary phase, while the maximum of interference is eliminated by simple nonretention. For maximum efficiency, the flow velocity of the sample should be moderate.
The next step is washing. It is not systematic; it aims to eliminate interferences weakly retained. It is necessary to choose solvents of weak eluent forces (e.g., solution methanol/water) to elute only the interferents. This step for so-called mixed phases can be multiplied by acting alternatively on one of the mechanisms, for example, first washing with a weak eluent strength solution for our analytes and then a second washing by modifying the pH of the mobile phase. These multiple washes improve very clearly the cleanliness of the extract contributing to the quality of the analysis. It is recommended at the end of this step to dry the support to evaporate traces of washing solvent. This step improves the extraction yield.
The last step is elution. It is preferable to use the solvent with the lowest possible eluting force capable of driving all the molecules of interest, thus avoiding eluting strongly retained interferents. The choice of the solvent is also guided by its ease of evaporation or its compatibility with the following analytical technique. It must nevertheless be as effective as possible; its volume must be low so as to obtain a very important preconcentration factor. The flow rate of the solvent must be slow to promote elution.
Finally, SPE has taken an important place in the preparation of samples over the years [ 13 , 40 ]. The range of stationary phases and their packaging are regularly enriched. This extraction method makes it possible to easily extract compounds that are difficult to extract, because they are very polar, with organic solvents and that could therefore only be analyzed after a simple precipitation. In addition, its automation that exists in different forms promises its wide utilization in the future by many laboratories. [ 13 , 40 , 41 ].
3.2. Liquid-Liquid Extraction (LLE)
Liquid-liquid extraction (LLE) methods allow the transfer of a solute initially contained in a liquid phase to another immiscible liquid phase. They are commonly used in pharmacology/toxicology to purify and concentrate samples prior to chromatographic or other analyses [ 20 , 42 ]. Various physicochemical parameters govern the production of an LLE, specific to the solvents used and to the solutes to be extracted. The knowledge of certain properties of the solvent such as its miscibility with water, acidity constant, dielectric constant, dipole moment, density, volatility, and its toxicity will allow the choice of this solvent alone or in mixture for the extraction of a given substance. In the same way, the knowledge of the properties of the solute such as the structure, the acidity constant, the lipophilic, the nature, and the complexity of the matrix in which it is will make it possible to optimize the extraction, whose efficiency will be evaluated by the extraction yield. The mastery of all these variables will allow the operator to optimize the LLE steps when developing analysis methods in pharmacology/toxicology.
4. Methods Commonly Used for the Analysis of BZDs in Biological Specimens
Many methods for the determination of BZDs in biological samples have been reported in the literature. Some are qualitative and some others are quantitative methods. Indeed, developed methods are classified as chromatography (HPLC, TLC, and GC) [ 11 – 15 , 18 ], immunoassays [ 38 , 43 – 48 ], photometric (nuclear magnetic resonance and ultraviolet-visible) [ 20 , 42 ], and electroanalytical methods (potentiometric, polarographic, and voltammetry) [ 49 – 51 ] have been increasingly used for drugs of abuse in forensic science.
Despite the fact that chromatographic methods still play a main role [ 52 ] in the detection and determination of BZDs in biological matrices [ 11 , 12 , 14 , 15 , 18 ], alternative methods like immunoassays [ 38 , 53 , 54 ] have been increasingly used for drugs of abuse in forensic science.
In case of suspected misuse or acute intoxication by BZDs, the toxicological analysis is often very useful for confirmation. The latter consists of three steps:
Step 1 . Screening using immunoenzymological methods, which allow a quick identification of the class of the offending drug
Step 2 . Identification by spectroscopic and/or chromatographic techniques, well adapted to the emergency but having certain limits
Step 3 . Quantification of the BZDs molecule by chromatographic or spectroscopic adapted techniques
To get accurate and reliable results, relatively clean samples need to be analyzed. As a result, the pretreatment of the biological sample is an essential part of any analytical method. It allows improving the reproducibility of the analysis, lowering the limit of quantification of the method by decreasing background noise and concentration steps, improving the fidelity and accuracy of the analysis, and finally increasing the selectivity.
In this regard, modern isolation techniques, e.g., solid-phase microextraction (SPME) or LLE [ 20 ] which help to concentrate volatile or nonvolatile compounds in samples before GC or HPLC analysis [ 13 , 20 ], solvent extraction after derivation, and stationary phases of grafted silica polar, are very important.
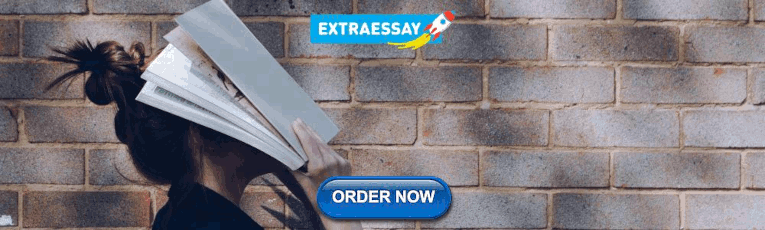
4.1. Screening/Detection Methods
The confirmation of BZDs consumption theoretically includes a screening test, followed, in case of positivity, by a confirmation test. Screening is usually done on company premises (workplaces) by “onsite” testing or in the laboratory by automated techniques. Overall, it is an immunological technique based on the recognition by a specific antibody (Ab) of the target BZD molecule (antigen) to be investigated. In these cases, biological media dedicated to screening are urine and saliva, although matrices such as blood or hair can be used.
There are currently many immunoenzymatic methods suitable for screening BZDs and their metabolites in body fluids. The principle lies in a competition between a labeled antigen and unlabeled antigen (BZD) against a specific antibody, and the major immunoassays are described below.
4.1.1. Lateral Flow Immunoassay (LFIA)
The lateral flow immunoassay is a rapid and a simple paper-based devices used for the detection of a target molecule in liquid sample without the need for costly equipment. This method offers an interesting detection level for BZDs screening with low circulating concentrations. For example, in 2014, Toubou et al. [ 45 ] used the Oratect® III commercialized LFIA to detect alprazolam, prazepam, diazepam, and estazolam in whole blood with a detection limit of 60, 75, 25, and 15 ng/mL, respectively, and Berck et al. [ 46 ] used an inhouse LFIA and found that the observed positive cutoff for oxazepam was 400 ng/ml. Over the past decade, there has been a growing interest in the use of these innovative devices and saliva testing, particularly in the area of road safety [ 10 , 43 , 44 ]. Indeed, there are indications that reliability of these tests is improving and number of countries using them for roadside checks is increasing. However, their use in occupational health remains very limited at the moment.
In rapid detection tests, there is no perfect correlation between the results of drug tests in saliva, blood, or urine analysis. This may due to the difference in time between the moment of drug consumption and the testing time. For example, in 2013, Mohamed et al. [ 47 ] found that tobacco interferes with laminar flow immunoassays (LFIA) of urinary drug detection; therefore, all subjects must be questioned about their smoking status to avoid false positive results. Oral fluid cannot be seen as a substitute for blood or urine drug testing. Each specimen has its own distinct advantages and disadvantages. For example, if a driver consumed the medication just before the test, he or she could display a positive result on a blood or a saliva test, but not on a urine test. On the other hand, if he or she is a drug abuser before the test, he or she could show a positive result only to a urine test. Thus, drug-screening challenge arises not in the screening devices but rather in determining the best ways to proceed with impairments. Most of commercialized drug detection rapid tests use a LFIA based on four variables:
The threshold, the level of concentration from which the device is able to detect a BZD.
The sensitivity of the device or the test refers to the probability of obtaining a positive result for the presence of drugs in the body at the time of analysis. Indeed, the higher the sensitivity of the device, the lower the false negative rate.
The specificity, the measure of the probability of getting a negative result, but no drugs in the body of the driver at the time of the test. The higher the specificity of the device, the lower the false positive rate. A screening device designed for use in workplaces with high levels of sensitivity and specificity makes it possible to quickly identify people who have used drugs and to minimize the detention time of people without drugs in their homes or organization.
The last variable is related to the biological detection medium.
4.1.2. Radioimmunoassay (RIA)
The radioimmunoassay (RIA) technique, as the name implies, achieves sensitivity through the use of radionuclides and specificity that is uniquely associated with immunochemical reactions. RIA is based on the competition between two antigens, which can bind to the same antibody. The radiolabeled, added in excess, and unlabeled antigens (tested molecule) compete for the limited binding sites on the antibody. The more the sample antigen is present, the less the radiolabeled antigen is able to bind to the antibody. The radiolabeled antigen must generally be present in low concentrations, because the quantity of molecule to be measured is usually small. Since the complex Ag-Ab is heavier than the one containing the unbound antigen, a centrifugation of the mixture will allow the separation into “free” and “bound” fractions and their radioactive counts measured. The concentration of test antigen can be calculated from the ratio of the bound and total antigen labels using a standard dose response curve. By measuring the radioactivity of the pellet, it is possible to determine the amount of radiolabeled Ag that has bound to Ab and therefore the concentration of Ag in the sample. Several authors have used this technique for the detection and quantification of benzodiazepines [ 55 ]. The most used isotopes in RIA are 3 H, 14 C, 32 P, 125 I, and 57 Co. However, because of the long half-life of the first three and because their disintegration passes by the emission of beta particles, only 125 I and 57 Co are still used, with a clear preference for 125 I, whose half-life is 60 days and emits easily detectable gamma particles. RIA technique is known for a low level of detection up to very low concentrations and high specificity. Although highly suitable for large series, the use of RIA is very rare in clinical laboratories, especially with the presence of enzyme-linked immunosorbent assay (ELISA) [ 56 ] and mainly because of the disadvantages inherent to the handling of radioisotopes. The majority of RIA assay formats recommend sample cleaning and concentration (particularly when analyte's concentration and assay sensitivity are low) [ 55 ].
4.1.3. Competitive Enzyme-Linked Immunosorbent Assay (ELISA)
The enzyme-linked immunosorbent assay (ELISA) technique is an immunoenzymatic detection and quantification technique that makes possible to visualize an antigen-antibody reaction by means of a color reaction produced by the action on a substrate of an enzyme previously fixed to the antibody. ELISA has been used in many studies to detect BZDs in different biological specimens [ 24 , 48 , 57 – 59 ]. There are several different ELISA methods, namely, the competitive and the noncompetitive ELISA. Usually, BZDs screening is done by the competitive ELISA assays that is frequently used for the detection of small antigens containing a single epitope. The competition occurs between labeled (in known quantity) and unlabeled antigen of interest (BZDs) for a limited number of antibody sites. The signal generated by this assay will be inversely proportional to the concentration of unlabeled antigen in the sample which could be quantified using the standard curve which is prepared by performing a dilution series of a known concentration of the analyte across a range of concentrations near the expected unknown concentration. There are systems for increasing the sensitivity, which means to reduce the detection threshold of the constituents by using substrates giving a larger signal for the same amount of enzyme, for example, using conjugates with β -galactosidase revealed with a fluorogenic substrate or peroxidase conjugates with emission of a flash of light revealed by chemiluminescence.
The second approach is to amplify the signal by increasing the amount of enzyme in the Ag-Ab-enzyme complex. But since it is impossible to prepare more marked conjugates (with more enzyme molecules) at the risk of denaturing the activity of the Ab or enzymes, it is resorted to couplings allowing real scaffolding, as the avidin-biotin. Each stage of the scaffold amplifies the preceding one.
This test offers an interesting detection of BZDs with low concentrations; for example, in 2015, O'Connor et al. [ 48 ] have used Immunalysis® Benzodiazepine ELISA kit to test the crossreactivity with 10 BZDs including etizolam, nitrazepam, 7-aminoflunitrazepam, oxazepam, temazepam, chlorodiazepoxide, diazepam, phenazepam, desmethyldiazepam, and lorazepam in a sample blood. The pyrazolam and etizolam had a limit of detection of 0.0025 mg/L and a limit of quantitation of 0.005 mg/L.
4.1.4. Cloned-Enzyme Donor Immunoassay (CEDIA)
Cloned-enzyme donor immunoassay for BZDs analysis is a single homogeneous phase immunoenzymatic method that uses recombinant DNA technology. This test uses the bacterial enzyme β -galactosidase previously split into two inactive fragments by genetic engineering. These fragments spontaneously reassociate to form a fully active enzyme that, upon reaction, fragments a substrate, producing a color change that can be measured by spectrophotometry. The drug (BZD) in the sample competes with the drug conjugated to one of the inactive fragments of β -galactosidase to bind to the Ab binding sites. If the drug is present in the sample, it attaches to the Ab, leaving the inactive fragments of the enzyme to form an active enzyme. If the sample does not contain a drug, the Ab binds to the conjugated drug of the inactive segment, hindering the reassociation of the inactive β -galactosidase fragments, which prevents the formation of an active enzyme. The amount of active enzyme formed and the resulting extinction variation are proportional to the amount of drug present in the sample. In order to improve the sensitivity of the test, an optional enzyme is added to hydrolyze the glycoconjugate metabolites of BZDs, thus favoring the detection of samples containing BZDs metabolites [ 38 , 53 , 54 , 60 – 64 ].
Every laboratory has to validate the CEDIA test to use depending on the requirements individually and define cutoff values, for example, Musshoff et al. in 2013 [ 54 ] and Darragh et al. in 2014 [ 38 ] after they have optimized the set of samples (urine), the cutoff of the assays was 25 and 200 ng/ml, respectively.
4.1.5. Fluorescence Polarization Immunoassay (FPIA)
The fluorescence polarization immunoassay uses the fluorescence polarization measurement emitted after excitation of a fluorescent substance by an equally polarized light beam. The degree of polarization of the emitted fluorescence directly depends on the amount of labeled ligand attached to the Ab. The sensitivity of FPIA technology is comparable to that of enzyme multiplied immunoassay technique (EMIT) methods. However, it has the main drawback of an unsuitable detection threshold for the lowest dose of BZDs especially triazolam and flunitrazepam, which are generally the most toxic ones. In fact, the results are positive only with toxic concentrations of BZDs, because a positive result is obtained for a concentration greater than 100 ng/ml, while the toxic concentrations of BZDs varied between 50 and 100 ng/ml [ 53 ]. From this point of view, the FPIA method has been less used in recent works.
4.1.6. Kinetic Interaction of Microparticles in Solution (KIMS)
The kinetic interaction of microparticles in solution (KIMS) test is based on the kinetic interaction of microparticles in a given solution. For example, Abs recognizing BZDs are covalently bound to microparticles, and the drug derivative is linked to a macromolecule. The kinetic interaction of the microparticles in the solution is induced by the binding of the drug conjugate to the Ab on the microparticles and inhibited by the presence of BZDs in the sample. The drug conjugate and the BZDs in the sample compete for the binding sites of the BZDs Abs to the microparticles. The resulting kinetic interaction of microparticles is indirectly proportional to the amount of drug present in the sample [ 38 ].
The KIMS assay was performed by Darragh et al. [ 38 ] according to manufacturer instructions and optimized to a cutoff of 100 ng/mL, a sensitivity of 47%, and a specificity of 100%, although Bertol et al. in 2013 [ 53 ] validated the KIMS assay to a cutoff of 200 ng/mL, a sensitivity of 100%, and a specificity of 40%. Generally, every laboratory optimizes its test to use according to the personal needs.
4.2. Identification and Quantification Methods
Although screening tests are very important for BZDs analysis, they offer only a provisional result. Using any immunological technique, the risk of false positive by cross reaction with another drug is important, and therefore, it requires confirmation of positive samples by a quantitative method such as chromatographic or spectroscopic allowing unambiguous identification and exact determination of the concentrations of the BZD molecule. The biological specimen dedicated for BZDs confirmation remains the blood, but recent work describes potential effective confirmation techniques using saliva or urine [ 11 , 12 , 14 , 17 – 19 ].
4.2.1. Gas Chromatography Coupled to Mass Spectrometry (GC-MS)
The gas chromatography coupled to the mass spectrometry (GC-MS) method is generally considered among the reference methods for BZDs detection and quantification. This technique is the most powerful tool for identifying such drugs in biological media because of its high sensitivity and specificity. The actual analysis by GC-MS is preceded by a step of sample preparation. This step is long and difficult to be automatable. Hence, the identification is based on the specific detection of high mass ions of the substance to be analyzed. The search for the identity of the specific ions obtained is performed by comparison with a given reference library [ 10 , 13 , 15 , 18 , 60 ].
GC-MS offers the double advantage of quantification and formal identification of BZDs with often low detection limits ( Table 1 ). In addition, the use of GC poses a number of problems mainly related to the thermolability of most BZDs that degrade rapidly in the absence of prior derivatization. However, this stage, often long and delicate, is only poorly suited to emergency toxicological analysis. Despite these difficulties, the GC-MS remains an interesting method when it comes to confirming an ambiguous diagnosis.
Usual limits of detection (LOD) and quantification (LOQ) of benzodiazepines by gas chromatography (GC-MS).
∗ Diazepam, nordazepam, oxazepam, bromazepam, alprazolam, lorazepam, medazepam, flurazepam, fludiazepam, tetrazepam, chlordiazepoxide, clobazam, midazolam, flunitrazepam, 7-aminoflunitrazepam, triazolam, prazepam, nimetazepam, temazepam, lormetazepam, clonazepam, and camazepam. ∗∗ 7-Aminoclonazepam, α –OH–alprazolam, α –OH–midazolam, alprazolam, bromazepam, clonazepam, diazepam, flunitrazepam, lorazepam, midazolam, nitrazepam, nordazepam, oxazepam, temazepam, and triazolam. ∗∗∗ The European Union project Driving under the Influence of Drugs (DRUID).
4.2.2. High-Performance Liquid Chromatography (HPLC)
HPLC is the technique of choice for BZDs analysis and quantification [ 12 , 65 ]. Compared with the GC method, HPLC does not expose molecules to thermal degradation and thus makes it possible to overcome derivatization reactions. A simple sample preparation step (liquid-liquid or solid-phase extraction) is usually sufficient. While detection by UV spectrometry is the most commonly used, coupling with mass spectrometry (HPLC-MS or MS-MS) is the method of choice for the identification and quantification of BZDs [ 11 , 12 , 60 ]. This method offers a particularly interesting detection level for the characterization and the determination of BZDs with very low circulating concentrations ( Table 2 ). In addition, the automation of extraction procedures, particularly in the solid phase, generally allows the analysis in a very short time, compatible with the emergency.
Usual limits of detection (LOD), quantification (LOQ), and cutoff of some benzodiazepines by HPLC.
4.2.3. Thin-Layer Chromatography (TLC)
The thin-layer chromatography (TLC) is the simplest of the chromatographic methods. Indeed, it consists in placing on a sheet (paper, silica, or others) a stain and let it elute by soaking in a recommended solvent or a mixture of solvents (called eluent). The eluent diffuses along the support. After that, the stain migrates on the leaf, more or less quickly depending on the nature of the interactions, it undergoes on the part of the support and the eluent. The revelation is then done by colored reactions. The TLC method is much less used in emergency cases in toxicology. This technique can be quite fast (about half an hour) but lacks specificity and sensitivity and especially the interpretation is delicate [ 66 , 67 ].
4.2.4. Ultraviolet-Visible Spectroscopy
The molecules of BZD absorb in ultraviolet (UV)/visible rays that can be assayed by this property under well-standardized conditions to be able to apply the law of Beer–Lambert. Each molecule of BZD has an absorption maximum at which the measurement will be carried out. Prior extraction and calibration range are required for each assay. It is necessary that the molecule to be assayed has a characteristic spectrum and that there are not in the biological medium other molecules extracted under the same conditions and absorbing in the same ranges of wavelengths. The wavelength chosen for the BZD assay is generally between 210 and 350 nm. The ease of identification of a substance by its ultraviolet or visible spectrum depends on not only the number of bands present in the spectrum but also the width of these bands (a narrow band usually has a clearer maximum) or the possibility for a band to appear as a shoulder on a band more intense. Some authors have demonstrated that benzodiazepines extracted from samples can be detected using this optimized technique; for example, Doctor and McCord in 2013 [ 20 ] have demonstrated that this method overcomes the limitations of some immunoassay tests by providing lower LOD ranging (from 0.5 ng/mL to 127 ng/mL) and giving reproducible results over a wide range of concentrations of eleven different benzodiazepines and metabolites, except flunitrazepam and chlordiazepoxide where the LOD was below 50 ng/mL.
Generally, the use of this technique for BZDs characterization is much less appreciated, compared to other techniques; only some laboratories use this technique.
4.2.5. Nuclear Magnetic Resonance Spectroscopy (NMR)
Nuclear magnetic resonance (NMR) spectroscopy is one of the most powerful techniques and versatile tools for retrieving detailed information about the structure, dynamics, and interactions of both organic and inorganic drugs. This technique has also proved to be useful in the qualitative and quantitative determination of the absorbing species such as BZD. Indeed, a study reported that Metizolam was detectable by NMR in hydrolyzed urine during the 46 hours period, with concentrations always lower than 11 ng/mL [ 42 ]. The resulting NMR spectra have very high information content, enabling the rapid detection and identification of analytes present in the sample such as urine [ 42 ]. Another favorable feature of NMR spectroscopy is that it is nondestructive, permitting the subsequent reanalysis of the sample by other methods [ 68 ].
There are different NMR spectroscopy experiments according to the studied nucleus and the type of information sought. We find NMR spectroscopy of 1 H, 2 H, 13 C, 15 N, 31 P (the common nuclei studied using NMR in biomedical research), 19 F, 17 O, etc. Likewise, the advent of novel technologies for NMR spectrometers allows henceforth the routine acquisition of sophisticated one-dimensional (1D) and two-dimensional (2D) NMR spectra in relatively short periods of time on complex molecules [ 68 ].
The NMR experiments must be carried out under the same physicochemical conditions, since the various parameters are variable according to the temperature and the pH of the solutions. Each NMR signal is characterized by several magnitudes that are characteristic of the considered nucleus. These parameters give information about the number of nuclei, their environment, and their connectivity [ 68 ].
4.2.6. Polarography
Polarography is a steady-state indicator method using intensity-potential curves plotted on a drop electrode of mercury. The solution transport of electroactive mercury species is due to diffusion. Following are the conditions of polarography:
Half-wave potentials are the characteristic of the electroactive substance, hence the possibility of qualitative analysis
The height of the bearings is proportional to the concentrations of these substances, hence the possibility of quantitative analysis
The conventional polarography has the main disadvantage of generating large capacitive currents due to the application of a potential to the electrode throughout the growth of the drop. This problem can be overcome by reducing the duration of application of the electrode potential used for current measurement, hence the idea of imposing short-term potential pulses [ 49 ].
There are two main versions of impulsion polarography: normal (PIN) and differential (PID). In PIN, from a constant value of potential, chosen in such a way that no electrochemical reaction takes place, pulses of variable amplitude are superimposed and incremented regularly from 1 to 2 mV so as to scan the potential area of interest. In PID, the amplitude of the pulse remains constant and it is the continuous potential that is incremented at each drop time to explore the window of potential interesting [ 49 ].
The main examples of applications include the following [ 50 ]:
Trace analysis in the environmental field (heavy metals and organic pollutants)
Analysis of bath components in the surface treatment industry (metals, additives, reducing agents, and impurities)
Analysis of drugs or active ingredients in the pharmaceutical industry (vitamins, steroids, antibiotics, psychotropic drugs (1, 4 BZDs)) especially in the formulated products and also in the blood, serum, urine, and plasma.
Analysis and quality control of food matrices such as ascorbic acid and vitamins and also contaminating compounds such as antibiotics, certain pesticides (Parathion), toxins (aflatoxin), or metals.
Analysis and control in the plastics and polymers industry including additives used.
4.2.7. Voltammetry
Voltammetry refers to the study of the intensity-potential curves of an electrochemical system. In this technique, a variable potential difference is applied across two electrodes of a measuring cell and the current flowing through the circuit is recorded. The cell contains a solution with chemical species that can give an oxidation or reduction reaction. Recent advances in electronics are at the basis of the development of this method, with the appearance of high-performance, highly sensitive equipment at affordable prices. Alone or coupled with other analytical techniques such as HPLC, GC, and TLC, it is used for the determination, qualitative or quantitative, of many organic substances like BZD [ 51 , 69 – 71 ].
The variation of the potential imposed on the working electrode, as a function of time, can be linear continuous, differential with pulse, or in the form of square signal. The curves obtained, called voltammograms, are at the base of all the other electrochemical methods: amperometry, potentiometry, and coulometry.
According to Naggar et al. in 2012 [ 72 ], the use of sonogel-carbon electrode modified with 5% bentonite in comparison with other electrodes used in voltammetric determination of 1,4-benzodiazepines and with chromatographic and spectrophotometric methods, it can be considered as a time-saving procedure, low cost, selective, and sensitive, because the quantification and detection limits were calculated as 6.0 and 19.5 ng/mL for diazepam and chlordiazepoxide hydrochloride, respectively. And, according to Panahi et al. in 2018 [ 73 ], to improve the selectivity of the electrochemical techniques, the modification of the working electrode with selective adsorbate materials to selectively uptake the target species on the electrode surface is a very good suggestion.
4.2.8. Potentiometry
Potentiometry is a measurement technique that passively evaluates the potential of a solution between two electrodes while affecting the solution in a minimal way. One of the electrodes is called the reference electrode (its potential remains constant), while the potential of the second (the working electrode) changes depending on the composition of the sample. The potential difference between the two electrodes then makes it possible to evaluate the composition of the sample with BZD [ 74 – 77 ].
The potentiometric technique generally involves working electrodes made selective for an ion of interest, so that the potential depends only on the activity of this ion of interest. The most widely used potentiometric electrode is the glass membrane electrode used in pH meters.
A variant of potentiometry is chronopotentiometry. This method consists in applying a constant current and measuring the potential as a function of time.
5. Conclusion
Various biological specimens and analysis methods have been conjointly used for the detection and quantification of psychotropic drugs, as described in this review. However, the most used biological samples are saliva, blood, and urine, whereas the commonly used analysis methods remain the quantitative and qualitative ones including HPLC and GC-MS. Toxicological screening using immunological tests, allowing the search for drugs such as BZDs, becomes more and more popular recently. The later tests will never replace those of the confirmation methods (HPLC, GC-MS, etc.), but they are complementary to establish a better and accurate diagnosis.
There is no ideal technique; hence, there is the need to choose several complementary methods according to the strategy adopted in each laboratory.
The choice of the toxicologist analyst should focus on separative methods, but it depends mainly on the vocation of his laboratory, priorities defined by the services local clinics, and technical and economic constraints in equipment and personnel. This situation requires a permanent dialogue between the doctor and the toxicologist analyst for optimal screening and follow-up.
Acknowledgments
We thank all members of the medical biotechnology for their kindness and support. We are also grateful to MASCIR for its support. This work was funded by the Moroccan ministry of transport, equipment and logistics through the National Center for Scientific and Technical Research (CNRST), as part of the “Programme d'appui à la recherche scientifique en matière de sécurité routière” (contract no. 21/2017).
Contributor Information
Zineb Qmichou, Email: [email protected].
Hassan Sefrioui, Email: [email protected].
Conflicts of Interest
The authors declare that they have no conflicts of interest in relation to this article.
- 1. Wick J. Y. The history of benzodiazepines. The Consultant Pharmacist. 2013;28(9):538–548. doi: 10.4140/tcp.n.2013.538. [ DOI ] [ PubMed ] [ Google Scholar ]
- 2. Offidani E., Guidi J., Tomba E., Fava G. A. Efficacy and tolerability of benzodiazepines versus antidepressants in anxiety disorders: a systematic review and meta-analysis. Psychotherapy and Psychosomatics. 2013;82(6):355–362. doi: 10.1159/000353198. [ DOI ] [ PubMed ] [ Google Scholar ]
- 3. Anthierens S., Pasteels I., Habraken H., Steinberg P., Declercq T., Christiaens T. Barriers to nonpharmacologic treatments for stress, anxiety, and insomnia: family physicians’ attitudes toward benzodiazepine prescribing. Canadian Family Physician. 2010;56(11):e398–e406. [ PMC free article ] [ PubMed ] [ Google Scholar ]
- 4. Naloto D. C. C., Lopes F. C., Barberato Filho S., Lopes L. C., Del Fiol F. d. S., Bergamaschi C. d. C. Prescrição de benzodiazepínicos para adultos e idosos de um ambulatório de saúde mental. Ciência and Saúde Coletiva. 2016;21(4):1267–1276. doi: 10.1590/1413-81232015214.10292015. [ DOI ] [ PubMed ] [ Google Scholar ]
- 5. Olfson M., King M., Schoenbaum M. Benzodiazepine use in the United States. JAMA Psychiatry. 2015;72(2):136–142. doi: 10.1001/jamapsychiatry.2014.1763. [ DOI ] [ PubMed ] [ Google Scholar ]
- 6. Gallacher J., Elwood P., Pickering J., Bayer A., Fish M., Ben-Shlomo Y. Benzodiazepine use and risk of dementia: evidence from the Caerphilly Prospective Study (CaPS) Journal of Epidemiology and Community Health. 2012;66(10):869–873. doi: 10.1136/jech-2011-200314. [ DOI ] [ PubMed ] [ Google Scholar ]
- 7. Griffin C. E., Kaye A. M., Bueno F. R., Kaye A. D. Benzodiazepine pharmacology and central nervous system–mediated effects. Ochsner Journal. 2013;13(2):214–223. [ PMC free article ] [ PubMed ] [ Google Scholar ]
- 8. Breggin P. R. Psychiatric drug-induced chronic brain impairment (CBI): implications for long-term treatment with psychiatric medication. International Journal of Risk and Safety in Medicine. 2011;23(4):193–200. doi: 10.3233/JRS-2011-0542. [ DOI ] [ PubMed ] [ Google Scholar ]
- 9. Furugen A., Nishimura A., Kobayashi M., Umazume T., Narumi K., Iseki K. Quantification of eight benzodiazepines in human breastmilk and plasma by liquid-liquid extraction and liquid-chromatography tandem mass spectrometry: application to evaluation of alprazolam transfer into breastmilk. Journal of Pharmaceutical and Biomedical Analysis. 2019;168:83–93. doi: 10.1016/j.jpba.2019.02.011. [ DOI ] [ PubMed ] [ Google Scholar ]
- 10. Pehrsson A., Blencowe T., Vimpari K., Langel K., Engblom C., Lillsunde P. An evaluation of on-site oral fluid drug screening devices DrugWipe® 5+ and rapid STAT® using oral fluid for confirmation analysis. Journal of Analytical Toxicology. 2011;35(4):211–218. doi: 10.1093/anatox/35.4.211. [ DOI ] [ PubMed ] [ Google Scholar ]
- 11. Melanson S. E. F., Griggs D., Bixho I., Khaliq T., Flood J. G. 7-aminoclonazepam is superior to clonazepam for detection of clonazepam use in oral fluid by LC-MS/MS. Clinica Chimica Acta. 2016;455:128–133. doi: 10.1016/j.cca.2016.01.027. [ DOI ] [ PubMed ] [ Google Scholar ]
- 12. Di Corcia D., Lisi S., Pirro V., Gerace E., Salomone A., Vincenti M. Determination of pharmaceutical and illicit drugs in oral fluid by ultra-high performance liquid chromatography-tandem mass spectrometry. Journal of Chromatography B. 2013;927:133–141. doi: 10.1016/j.jchromb.2013.01.025. [ DOI ] [ PubMed ] [ Google Scholar ]
- 13. Karlonas N., Padarauskas A., Ramanavicius A., Ramanaviciene A. Mixed-mode SPE for a multi-residue analysis of benzodiazepines in whole blood using rapid GC with negative-ion chemical ionization MS. Journal of Separation Science. 2013;36(8):1437–1445. doi: 10.1002/jssc.201201069. [ DOI ] [ PubMed ] [ Google Scholar ]
- 14. Mercieca G., Odoardi S., Cassar M., Strano Rossi S. Rapid and simple procedure for the determination of cathinones, amphetamine-like stimulants and other new psychoactive substances in blood and urine by GC–MS. Journal of Pharmaceutical and Biomedical Analysis. 2018;149:494–501. doi: 10.1016/j.jpba.2017.11.024. [ DOI ] [ PubMed ] [ Google Scholar ]
- 15. Papoutsis I. I., Athanaselis S. A., Nikolaou P. D., Pistos C. M., Spiliopoulou C. A., Maravelias C. P. Development and validation of an EI–GC–MS method for the determination of benzodiazepine drugs and their metabolites in blood: applications in clinical and forensic toxicology. Journal of Pharmaceutical and Biomedical Analysis. 2010;52(4):609–614. doi: 10.1016/j.jpba.2010.01.027. [ DOI ] [ PubMed ] [ Google Scholar ]
- 16. Samanidou V., Kaltzi I., Kabir A., Furton K. G. Simplifying sample preparation using fabric phase sorptive extraction technique for the determination of benzodiazepines in blood serum by high-performance liquid chromatography. Biomedical Chromatography. 2016;30(6):829–836. doi: 10.1002/bmc.3615. [ DOI ] [ PubMed ] [ Google Scholar ]
- 17. Lee H. H., Lee J. F., Lin S. Y., et al. Simultaneous quantification of urine flunitrazepam, nimetazepam and nitrazepam by using liquid chromatography tandem mass spectrometry. Clinica Chimica Acta. 2013;420:134–139. doi: 10.1016/j.cca.2012.10.023. [ DOI ] [ PubMed ] [ Google Scholar ]
- 18. Perez E. R., Knapp J. A., Horn C. K., Stillman S. L., Evans J. E., Arfsten D. P. Comparison of LC–MS–MS and GC–MS analysis of benzodiazepine compounds included in the drug demand reduction urinalysis program. Journal of Analytical Toxicology. 2016;40(3):201–207. doi: 10.1093/jat/bkv140. [ DOI ] [ PMC free article ] [ PubMed ] [ Google Scholar ]
- 19. Schaefer N., Peters B., Schmidt P., Ewald A. H. Development and validation of two LC-MS/MS methods for the detection and quantification of amphetamines, designer amphetamines, benzoylecgonine, benzodiazepines, opiates, and opioids in urine using turbulent flow chromatography. Analytical and Bioanalytical Chemistry. 2013;405(1):247–258. doi: 10.1007/s00216-012-6458-9. [ DOI ] [ PubMed ] [ Google Scholar ]
- 20. Doctor E. L., McCord B. Comparison of aggregating agents for the surface-enhanced Raman analysis of benzodiazepines. The Analyst. 2013;138(20):5926–5932. doi: 10.1039/c3an00669g. [ DOI ] [ PubMed ] [ Google Scholar ]
- 21. Moretti M., Andrello L., Visonà S., et al. Evaluation of benzodiazepines and zolpidem in nails and their stability after prolonged exposure to chlorinated water. Journal of Pharmaceutical and Biomedical Analysis. 2018;152:137–142. doi: 10.1016/j.jpba.2018.01.051. [ DOI ] [ PubMed ] [ Google Scholar ]
- 22. Lee S., Han E., In S., Choi H., Chung H., Chung K. H. Determination of illegally abused sedative-hypnotics in hair samples from drug offenders. Journal of Analytical Toxicology. 2011;35(5):312–315. doi: 10.1093/anatox/35.5.312. [ DOI ] [ PubMed ] [ Google Scholar ]
- 23. Marin S. J., Merrell M., McMillin G. A. Drugs of abuse detection in meconium: a comparison between ELISA and biochip microarray. Journal of Analytical Toxicology. 2011;35(1):40–45. doi: 10.1093/anatox/35.1.40. [ DOI ] [ PubMed ] [ Google Scholar ]
- 24. Wang J., Wang Y., Pan Y., et al. Preparation of a broadly specific monoclonal antibody-based indirect competitive ELISA for the detection of benzodiazepines in edible animal tissues and feed. Food Analytical Methods. 2016;9(12):3407–3419. doi: 10.1007/s12161-016-0528-0. [ DOI ] [ Google Scholar ]
- 25. Beck O., Stephanson N., Sandqvist S., Franck J. Detection of drugs of abuse in exhaled breath using a device for rapid collection: comparison with plasma, urine and self-reporting in 47 drug users. Journal of Breath Research. 2013;7(2) doi: 10.1088/1752-7155/7/2/026006.026006 [ DOI ] [ PubMed ] [ Google Scholar ]
- 26. Vindenes V., Lund H. M. E., Andresen W., et al. Detection of drugs of abuse in simultaneously collected oral fluid, urine and blood from Norwegian drug drivers. Forensic Science International. 2012;219(1–3):165–171. doi: 10.1016/j.forsciint.2012.01.001. [ DOI ] [ PubMed ] [ Google Scholar ]
- 27. Kintz P. Traité de Toxicologie Médico-Judiciaire. Amsterdam, Netherlands: Elsevier Health Sciences; 2012. [ Google Scholar ]
- 28. Drummer O. Urine and oral fluid in workplace drug testing. TIAFT Bulletin. 2008;38(2):39–40. [ Google Scholar ]
- 29. Bosker W. M., Huestis M. A. Oral fluid testing for drugs of abuse. Clinical Chemistry. 2009;55(11):1910–1931. doi: 10.1373/clinchem.2008.108670. [ DOI ] [ PMC free article ] [ PubMed ] [ Google Scholar ]
- 30. Substance Abuse and Mental Health Services Administration. Clinical Drug Testing in Primary Care. Technical Assistance Publication (TAP) 32 HHS Publication No. (SMA) 12-4668. Rockville, MD, USA: US Department of Health and Human Services. Substance Abuse and Mental Health Services Administration; 2012. [ Google Scholar ]
- 31. Townsend S., Fanning L., O’Kennedy R. Salivary analysis of drugs—potential and difficulties. Analytical Letters. 2008;41(6):925–948. doi: 10.1080/00032710802053815. [ DOI ] [ Google Scholar ]
- 32. Toennes S. W., Steinmeyer S., Maurer H.-J., Moeller M. R., Kauert G. F. Screening for drugs of abuse in oral fluid—correlation of analysis results with serum in forensic cases. Journal of Analytical Toxicology. 2005;29(1):22–27. doi: 10.1093/jat/29.1.22. [ DOI ] [ PubMed ] [ Google Scholar ]
- 33. Drummer O. H. Review: pharmacokinetics of illicit drugs in oral fluid. Forensic Science International. 2005;150(2-3):133–142. doi: 10.1016/j.forsciint.2004.11.022. [ DOI ] [ PubMed ] [ Google Scholar ]
- 34. Verstraete A. Drogues et accidentalité. Les Ulis, France: EDP Sciences; 2011. Le dépistage salivaire; pp. 235–255. [ Google Scholar ]
- 35. Huestis M. A., Verstraete A., Kwong T. C., Morland J., Vincent M. J., de la Torre R. Oral fluid testing: promises and pitfalls. Clinical Chemistry. 2011;57(6):805–810. doi: 10.1373/clinchem.2010.152124. [ DOI ] [ PMC free article ] [ PubMed ] [ Google Scholar ]
- 36. Barbosa F., Jr., Corrêa Rodrigues M. H., Buzalaf M. R., Krug F. J., Gerlach R. F., Tanus-Santos J. E. Evaluation of the use of salivary lead levels as a surrogate of blood lead or plasma lead levels in lead exposed subjects. Archives of Toxicology. 2006;80(10):633–637. doi: 10.1007/s00204-006-0096-y. [ DOI ] [ PubMed ] [ Google Scholar ]
- 37. Morton J., Leese E., Harding A.-H., Jones K., Sepai O. Saliva as a matrix for biomonitoring of occupational and environmental exposure to lead. Biomonitoring. 2014;1(1) doi: 10.2478/bimo-2014-0008. [ DOI ] [ Google Scholar ]
- 38. Darragh A., Snyder M. L., Ptolemy A. S., Melanson S. KIMS, CEDIA, and HS-CEDIA immunoassays are inadequately sensitive for detection of benzodiazepines in urine from patients treated for chronic pain. Pain Physician. 2014;17(4):359–366. [ PubMed ] [ Google Scholar ]
- 39. Kadehjian L. Drugs of Abuse. Berlin, Germany: Springer; 2005. Specimens for drugs-of-abuse testing; pp. 11–28. [ Google Scholar ]
- 40. Fernández P., Vázquez C., Lorenzo R. A., Carro A. M., Bermejo A. M. Development of a liquid chromatographic method for the simultaneous determination of six benzodiazepines in human plasma after solid-phase extraction. Analytical Letters. 2010;43(6):1075–1084. doi: 10.1080/00032710903492490. [ DOI ] [ Google Scholar ]
- 41. Simonsen K. W., Hermansson S., Steentoft A., Linnet K. A validated method for simultaneous screening and quantification of twenty-three benzodiazepines and metabolites plus zopiclone and zaleplone in whole blood by liquid-liquid extraction and ultra-performance liquid chromatography-tandem mass spectrometry. Journal of Analytical Toxicology. 2010;34(6):332–341. doi: 10.1093/jat/34.6.332. [ DOI ] [ PubMed ] [ Google Scholar ]
- 42. Kintz P., Richeval C., Jamey C., et al. Detection of the designer benzodiazepine metizolam in urine and preliminary data on its metabolism. Drug Testing and Analysis. 2017;9(7):1026–1033. doi: 10.1002/dta.2099. [ DOI ] [ PubMed ] [ Google Scholar ]
- 43. Blencowe T., Pehrsson A., Lillsunde P., et al. An analytical evaluation of eight on-site oral fluid drug screening devices using laboratory confirmation results from oral fluid. Forensic Science International. 2011;208(1–3):173–179. doi: 10.1016/j.forsciint.2010.11.026. [ DOI ] [ PubMed ] [ Google Scholar ]
- 44. Musshoff F., Hokamp E. G., Bott U., Madea B. Performance evaluation of on-site oral fluid drug screening devices in normal police procedure in Germany. Forensic Science International. 2014;238:120–124. doi: 10.1016/j.forsciint.2014.02.005. [ DOI ] [ PubMed ] [ Google Scholar ]
- 45. Toubou H., Namera A., Arima Y., et al. Detection of abused drugs in human blood by using the on-site drug-screening device Oratect® III. Legal Medicine. 2014;16(5):308–313. doi: 10.1016/j.legalmed.2014.04.004. [ DOI ] [ PubMed ] [ Google Scholar ]
- 46. Beck O., Carlsson S., Tusic M., Olsson R., Franzen L., Hulten P. Laboratory and clinical evaluation of on-site urine drug testing. Scandinavian Journal of Clinical and Laboratory Investigation. 2014;74(8):681–686. doi: 10.3109/00365513.2014.939995. [ DOI ] [ PubMed ] [ Google Scholar ]
- 47. Mouhamed D. H., Ezzaher A., Neffati F., Douki W., Gaha L., Najjar M. F. 1989–False benzodiazepines results in a rapid immunochromatography test for urinary drug detection in tobacco smokers. European Psychiatry. 2013;28:p. 1. doi: 10.1016/s0924-9338(13)76926-8. [ DOI ] [ Google Scholar ]
- 48. O’Connor L. C., Torrance H. J., McKeown D. A. ELISA detection of phenazepam, etizolam, pyrazolam, flubromazepam, diclazepam and delorazepam in blood using ImmunalysisBenzodiazepine kit. Journal of Analytical Toxicology. 2015;40(2):159–161. doi: 10.1093/jat/bkv122. [ DOI ] [ PubMed ] [ Google Scholar ]
- 49. Miomandre F., Sadki S., Audebert P., Méallet-Renault R. Électrochimie-2e éd.: Des Concepts Aux Applications. Paris, France: Dunod; 2011. [ Google Scholar ]
- 50. Hauchard D. Polarographie-Principes d’application et mise en oeuvre des techniques polarographiques. Techniques de l’Ingénieur. 2011;2136:1–5. [ Google Scholar ]
- 51. Doménech-Carbó A., Martini M., de Carvalho L. M., Viana C., Doménech-Carbó M. T., Silva M. Standard additions-dilution method for absolute quantification in voltammetry of microparticles. Application for determining psychoactive 1,4-benzodiazepine and antidepressants drugs as adulterants in phytotherapeutic formulations. Journal of Pharmaceutical and Biomedical Analysis. 2013;80:159–163. doi: 10.1016/j.jpba.2013.03.005. [ DOI ] [ PubMed ] [ Google Scholar ]
- 52. Persona K., Madej K., Knihnicki P., Piekoszewski W. Analytical methodologies for the determination of benzodiazepines in biological samples. Journal of Pharmaceutical and Biomedical Analysis. 2015;113:239–264. doi: 10.1016/j.jpba.2015.02.017. [ DOI ] [ PubMed ] [ Google Scholar ]
- 53. Bertol E., Vaiano F., Borsotti M., Quercioli M., Mari F. Comparison of immunoassay screening tests and LC–MS-MS for urine detection of benzodiazepines and their metabolites: results of a national proficiency test. Journal of Analytical Toxicology. 2013;37(9):659–664. doi: 10.1093/jat/bkt063. [ DOI ] [ PubMed ] [ Google Scholar ]
- 54. Musshoff F., Wolters T., Lott S., Ippisch J., Gradl S., Madea B. Optimization and validation of CEDIA drugs of abuse immunoassay tests in serum and urine on an Olympus AU 400. Drug Testing and Analysis. 2013;5(5):366–371. doi: 10.1002/dta.1454. [ DOI ] [ PubMed ] [ Google Scholar ]
- 55. Grange R., Thompson J., Lambert D. Radioimmunoassay, enzyme and non-enzyme-based immunoassays. British Journal of Anaesthesia. 2014;12(2):213–216. doi: 10.1093/bja/aet293. [ DOI ] [ PubMed ] [ Google Scholar ]
- 56. Alhabbab R. Y. Basic Serological Testing. Berlin, Germany: Springer; 2018. Radioimmunoassay (RIA) pp. 77–81. [ Google Scholar ]
- 57. Guan D., Guo L., Liu L., Kong N., Kuang H., Xu C. Development of an ELISA for nitrazepam based on a monoclonal antibody. Food and Agricultural Immunology. 2015;26(5):611–621. doi: 10.1080/09540105.2014.998637. [ DOI ] [ Google Scholar ]
- 58. Kyle P. B., Brown K. B., Bailey A. P., Stevenson J. L. Reactivity of commercial benzodiazepine immunoassays to phenazepam. Journal of Analytical Toxicology. 2012;36(3):207–209. doi: 10.1093/jat/bks008. [ DOI ] [ PubMed ] [ Google Scholar ]
- 59. Shan W. C., Cui Y. L., He X., Zhang L., Liu J., Wang J. P. Production of monoclonal antibody against clonazepam for immunoassay of benzodiazepine drugs in swine tissues. Journal of Environmental Science and Health, Part B. 2015;50(1):15–22. doi: 10.1080/03601234.2015.964620. [ DOI ] [ PubMed ] [ Google Scholar ]
- 60. French D., Stone J. A., Chang J. S., Wu A. H. B. Choosing the right benzodiazepine assay: impact on clinical decision making. Laboratory Medicine. 2010;41(4):196–200. doi: 10.1309/lmdnlg1zvos2r8tv. [ DOI ] [ Google Scholar ]
- 61. Agius R., Nadulski T., Kahl H.-G., Dufaux B. Comparison of LUCIO®-direct ELISA with CEDIA immunoassay for zero tolerance drug screening in urine as required by the German re-licensing guidelines. Drug Testing and Analysis. 2013;5(6):390–399. doi: 10.1002/dta.1455. [ DOI ] [ PubMed ] [ Google Scholar ]
- 62. Beck O., Rausberg L., Al-Saffar Y., et al. Detectability of new psychoactive substances, “legal highs,” in CEDIA, EMIT, and KIMS immunochemical screening assays for drugs of abuse. Drug Testing and Analysis. 2014;6(5):492–499. doi: 10.1002/dta.1641. [ DOI ] [ PubMed ] [ Google Scholar ]
- 63. Kirschbaum K. M., Musshoff F., Schmithausen R., Stockhausen S., Madea B. Optimization and validation of CEDIA drugs of abuse immunoassay tests in serum on Hitachi 912. Forensic Science International. 2011;212(1–3):252–255. doi: 10.1016/j.forsciint.2011.06.029. [ DOI ] [ PubMed ] [ Google Scholar ]
- 64. Köhler K. M., Hammer R., Riedy K., Auwärter V., Neukamm M. A. Evaluation of CEDIA and DRI drugs of abuse immunoassays for urine screening on a Thermo Indiko Plus analyzer. Journal of Clinical Laboratory Analysis. 2017;31(1) doi: 10.1002/jcla.22021.e22021 [ DOI ] [ PMC free article ] [ PubMed ] [ Google Scholar ]
- 65. Piergiovanni M., Cappiello A., Famiglini G., Termopoli V., Palma P. Determination of benzodiazepines in beverages using green extraction methods and capillary HPLC-UV detection. Journal of Pharmaceutical and Biomedical Analysis. 2018;154:492–500. doi: 10.1016/j.jpba.2018.03.030. [ DOI ] [ PubMed ] [ Google Scholar ]
- 66. Shweta D., Kesharvani L., Gupta A. K., Mishra M. K. Analysis of suspected seized sample of NDPS drugs (benzodiazepines) through GLC and TLC using different solvent system. Indian Journal of Forensic Medicine and Toxicology. 2015;9(1):p. 151. [ Google Scholar ]
- 67. Thangadurai S., Dhanalakshmi A., Kannan M. V. S. Separation and detection of certain benzodiazepines by thin-layer chromatography. Malaysian Journal of Forensic Sciences. 2013;4(1):47–53. [ Google Scholar ]
- 68. McClure C. K. Structural chemistry using NMR spectroscopy, organic molecules. In: Lindon J. C., Tranter G. E., Koppenaal D. W., editors. Encyclopedia of Spectroscopy and Spectrometry (Third Edition) Oxford, UK: Academic Press; 2017. pp. 281–292. [ Google Scholar ]
- 69. Shahraki S., Ahmar H., Nejati-Yazdinejad M. Electrochemical determination of nitrazepam by switchable solvent based liquid-liquid microextraction combined with differential pulse voltammetry. Microchemical Journal. 2018;142:229–235. doi: 10.1016/j.microc.2018.07.003. [ DOI ] [ Google Scholar ]
- 70. Garcia-Gutierrez E., Lledo-Fernandez C. The electroanalytical sensing of flunitrazepam (rohypnol) and 7-amino flunitrazepam in oral fluid, urine and alcoholic beverages. Universal Journal of Chemistry. 2013;1:121–127. doi: 10.3390/chemosensors1030068. [ DOI ] [ Google Scholar ]
- 71. Samiec P., Navrátilová Z., Fischer J. Voltammetry of benzodiazepines on meniscus-modified silver solid amalgam electrode. Monatshefte für Chemie-Chemical Monthly. 2016;147(1):127–134. doi: 10.1007/s00706-015-1594-5. [ DOI ] [ Google Scholar ]
- 72. Naggar A. H., ElKaoutit M., Naranjo-Rodriguez I., El-Sayed A. E.-A. Y., de Cisneros J. L. H.-H. Use of a sonogel-carbon electrode modified with bentonite for the determination of diazepam and chlordiazepoxide hydrochloride in tablets and their metabolite oxazepam in urine. Talanta. 2012;89:448–454. doi: 10.1016/j.talanta.2011.12.061. [ DOI ] [ PubMed ] [ Google Scholar ]
- 73. Panahi Y., Motaharian A., Hosseini M. R. M., Mehrpour O. High sensitive and selective nano-molecularly imprinted polymer based electrochemical sensor for midazolam drug detection in pharmaceutical formulation and human urine samples. Sensors and Actuators B: Chemical. 2018;273:1579–1586. doi: 10.1016/j.snb.2018.07.069. [ DOI ] [ Google Scholar ]
- 74. Soroush S., Maryam B. Potentiometric determination of clonazepam using carbon paste electrode based on Molecular Imprinted Polymer (MIP) in solution and in a biological fluid model. Pharmaceutica Analytica Acta. 2016;7(7):p. 2. doi: 10.4172/2153-2435.1000499. [ DOI ] [ Google Scholar ]
- 75. Ghorbani N., Hosseinzadeh S., Pashaei S., Hosseinzadeh A. Preparation of unique potentiometric carbon paste sensor for determination of diazepam in pharmaceutical applications. International Journal of Electrochemical Science. 2014;9:7574–7586. [ Google Scholar ]
- 76. Ghorbani N., Hosseinzadeh S., Pashaei S., Hosseinzadeh A., Hamidi H. A. The effect of produce conditions for preparation of potentiometric carbon paste sensor for determination of midazolam in pharmaceutical. International Journal of Electrochemical Science. 2014;9:3772–3783. [ Google Scholar ]
- 77. Ganjali M. R., Larijani B., Norouzi P. Determination of midazolam by potentiometric PVC membrane and MWCNTS based carbon paste sensors. International Journal of Electrochemical Science. 2012;7:4822–4833. [ Google Scholar ]
- View on publisher site
- PDF (1.1 MB)
- Collections
Similar articles
Cited by other articles, links to ncbi databases.
- Download .nbib .nbib
- Format: AMA APA MLA NLM
Add to Collections
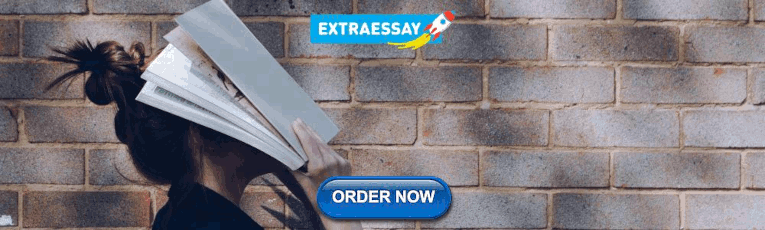
COMMENTS
Drug Testing and Analysis is the unrivaled specialist journal for drug testing practitioners. The journal provides a focal point for the detection of illicit and controversial substances, covering sports doping, recreational drugs, pharmaceuticals, toxicologic pathology, forensics, and the environment. Rather than focus on the application of a ...
Broadly defined, drug testing uses a biological sample to detect the presence or absence of a drug or its metabolites. This process can be completed in a variety of settings and with a variety of techniques. Many drug screening immunoassays were initially designed for use in the workplace as a drug screening tool for employees. As these tests have become cheaper, more readily available, and ...
A common characteristic of the above studies is that they use observational data to examine the nature of the cross-sectional association between workplace drug testing and worker drug use (we use a similar approach below). In contrast, we are aware of only one large-scale study to use a pre–post treatment-control research design to study the ...
Explore the latest full-text research PDFs, articles, conference papers, preprints and more on DRUG TESTING. Find methods information, sources, references or conduct a literature review on DRUG ...
American Society for Addiction Medicine. Drug Testing: A White Paper of the American Society of Addiction Medicine (ASAM). Chevy Chase, MD: 2013. Levy S, Siqueira LM, Ammerman SD, et al. Testing for drugs of abuse in children and adolescents. Pediatrics 2014;133:e1798–807. Substance Abuse and Mental Health Services Administration.
For example, it is well known that an individual who is a chronic user of marijuana can test positive for days or even weeks after having stopped using the drug, and that marijuana use that is completely divorced from the work setting can nevertheless lead to a positive drug test. Research on attitudes toward drug testing and on perceptions of ...
This led to the establishment of the first urine drug testing laboratory and the development of forensic drug testing standards and procedures (Gannon, 2014). In 1986, WDT was implemented when President Ronald Reagan gave the executive order to develop a method for keeping the workplace free of drug abuse Bush, 2008 , Willette et al., 1988 .
Urine drug tests are the most common types of tests used among medical professionals. Many countries adopted these tests for road control, and many jobs now require a screening in order to apply. In research area, many studies used urine as biological matrix for drug analysis [14, 17–20, 38]. Indeed, the search for illicit drugs in the urine ...
In a fast-evolving doping environment, where sport professionals are constantly seeking novel and illegal means to bypass doping tests, and new substances are regularly detected on the drug market, it is crucial to inform authorities with updated evidence emerging from scientific research.
Rather than focus on the application of a single technique, Drug Testing and Analysis employs a unique multidisciplinary approach to the field of controversial compound determination. Papers discussing chromatography, mass spectrometry, immunological approaches, 1D/2D gel electrophoresis, to name just a few select methods, are welcomed where ...