Fan Performance Characteristics at Various Rotational Speeds and Ambient Pressures 2014-01-2219
The scaling laws of fans express basic relationships among the variables of fan static pressure head, volume flow rate, air density, rotational speed, fan diameter, and power. These relationships make it possible to compare the performance of geometrically similar fans in dissimilar conditions. The fan laws were derived from dimensionless analysis of the equations for volumetric flow rate, static pressure head, and power as a function of fan diameter, air density and rotational speed. The purpose of this study is to characterize a fan's performance characteristics at various rotational speeds and ambient pressures. The experimental results are compared to the fan scaling laws.
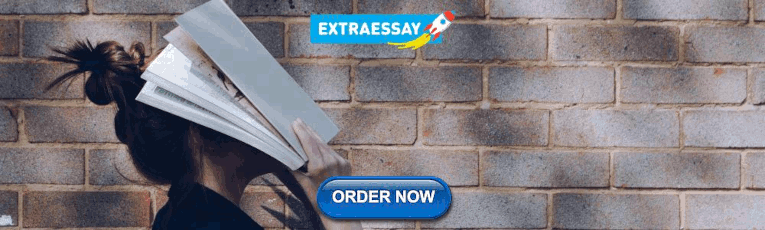
SAE MOBILUS
Subscribers can view annotate, and download all of SAE's content. Learn More »
Access SAE MOBILUS »
AST Propulsion Comparisons
View Details
Improved Thermal and Mechanical Design of Copper/Brass Radiators
Experimental Analysis for the Improvement of Radiator Cooling Air Intake and Discharge
Stack Exchange Network
Stack Exchange network consists of 183 Q&A communities including Stack Overflow , the largest, most trusted online community for developers to learn, share their knowledge, and build their careers.
Q&A for work
Connect and share knowledge within a single location that is structured and easy to search.
Fan Speed Formula
Is there any formula for computing fan speed by using air mass,air flux, air density or specific heat? I have computed air mass and air flux, and found the values for air density and specific heat, but now I am stuck at finding a correlation between these and fan speed.
- thermodynamics
- $\begingroup$ Can you reproduce your computing here much appreciated $\endgroup$ – user6760 Commented May 18, 2015 at 12:20
- $\begingroup$ I start from 2 equations for computing the power consumption of a data center: P = m Cp*(Tout-Tin) and P=p f*Cp(Tout-Tin), where m=air mass, Cp= specific heat, p=air density (1.205kg/m^3 at 20degreesC),f= air flow rate = m/p, Tin = the inlet temperature (temperature supplied by a CRAC unit), Tout=exhaust temperature. I also know that the fan power Pfan=(FanSpeed^3) (equation 3). What I need, is to find a correlation between the first 2 equation and the 3rd one $\endgroup$ – Ade Commented May 19, 2015 at 10:05
- $\begingroup$ The fan laws state that the air flow is proportional with the fan speed. So, basically, I need to convert airflow (m^3/s) to speed $\endgroup$ – Ade Commented May 19, 2015 at 13:54
2 Answers 2
From an engineering perspective, there are many different fan designs including axial and centrifugal configurations, along with various blade designs including forward curved, backward curved, and radial. There is no formula to calculate the required fan speed, but if a specific fan configuration is known then fan similarity laws can be used to calculate performance based on known performance of a similar fan. For example, for a given fan design the flow varies linearly with speed, static pressure varies with the square of the speed, and power consumption varies with the cube of the speed. Similarly, at the same speed flow varies with the cube of the impeller diameter, and static pressure varies as the square of the diameter.
These formulas are described here for example.
Using a dimensional analysis indeed can help you find dimensionless numbers as The Dark Side suggested Floris might help with, but beyond that there is no direct analytical method, no closed form solutions that can relate fan speed, shaft torque, flow rate and delta pressure. The issue is that at best the flow behavior is two dimensional, but more likely three dimensional in its behavior. There are two approach's to find the relationships:
(1) Do the dimensionless analysis, come up with dimensionless numbers, then do experiments with your fan to find the relationship of speed to the other parameters. Then you can fit the relationships to polynomials.
(2) Model the fan in CAD, then do a dimensional CFD simulation to relate your parameters of interest.
The difficulty in trying to obtain an analytical solution directly from the fundamental energy, momentum and continuity principles comes from the nonlinear nature of the Navier Stokes equations and the intractability of the equations in particular 2 dimensional and 3 dimensional problems. If fluid rotation can occur you are stuck doing 3 dimensional analysis. The other factor is the complex description of the fan's surface. Not all fans are alike.

Your Answer
Sign up or log in, post as a guest.
Required, but never shown
By clicking “Post Your Answer”, you agree to our terms of service and acknowledge you have read our privacy policy .
Not the answer you're looking for? Browse other questions tagged thermodynamics energy air cooling or ask your own question .
- Featured on Meta
- Preventing unauthorized automated access to the network
- Upcoming initiatives on Stack Overflow and across the Stack Exchange network...
- Our Help center policy article on generative AI content
Hot Network Questions
- Producing solo work
- Why does only the Septuagint have "Ca'inan" in the genealogy in Genesis 10 and 11?
- Diagonalisation in the proof of undecidability of the acceptance problem for Turing Machines
- Can a carbon crank be safely put into a vice?
- Inductive effect explanation with MO theory
- I'm trying to replicate Rømer's experiment but can't seem to get even close to the correct value for the speed of light
- Is philosophy of declining influence, effectively dead or irrelevant in modern times? If so, why?
- MySQL using a multi-column index even when the first column isn't being queried
- Why does my iPhone 16 Pro have iOS 18.0 but I can’t find a way to activate developer mode anywhere?
- Who are "the great Florentine" and "Mireya"?
- What is the relevance of mention of women in Jesus' Genealogy?
- Series of short stories about hillbillies who are actually aliens
- Soldiers bred for battle are killed when peace begins
- Accidentally drilled holes through dryer duct
- What is Iran's long-term objective in the Middle East?
- Should chat audio be encrypted before sending it?
- In Sadi's Bustan, is sweet wormwood Korean worm wood?
- What does "to eliminate the meddling middlemen of public accountability and free will" mean?
- When Mr. Incredible saved a man from killing himself, is he really liable for damages?
- What is the term for types that are not type variables?
- “I know what’s best but I do the opposite” translation?
- How can I draw a simple house?
- Every manifold can be cut into cubes
- SwiftUI TimeUnits-Converter App
Stack Exchange Network
Stack Exchange network consists of 183 Q&A communities including Stack Overflow , the largest, most trusted online community for developers to learn, share their knowledge, and build their careers.
Q&A for work
Connect and share knowledge within a single location that is structured and easy to search.
Fan speed and power consumption
Really basic question that I've struggled with for a while. In low-cost fans, a series of mechanical buttons select fan speed. I assume each button is connected to a specific resistor which limits the current/divides the voltage to the fan motor, depending on how they are arranged.
My question is, is it irrelevant to the overall power used by the appliance whether the fan is operating on low or high speed? My assumption is that the energy is simply wasted to heat the resistor on the low speed setting.
- induction-motor
2 Answers 2
These fans use shaded pole motors which are a type of asynchronous motor.
Speed can be controlled by varying winding current.
This isn't done with actual resistors, but by switching between several windings. The lowest speed setting corresponds to the longest length of wire in the windings, thus the highest resistance. Highest speed corresponds to lowest resistance (shortest winding). This can also be done by switches connecting windings in series, parallel, or a combination.

Also, more winding turns mean higher inductance, thus higer impedance, which reduces current without wasting power in winding resistance.
Since higher impedance results in lower current, the fan does not draw constant power from mains, and lower speeds do use less power.
- 1 \$\begingroup\$ As a side note, when the motor fails, it is often the winding between the common and the high connection. By disconnecting medium, and connecting high directly to common, you can get the fan to work for a short time, albeit faster than high, probably overheating the motor, and certainly not UL approved. The current runs only through the windings between the high connection and the low connection. I've extended the "fan life" by additional weeks doing this. \$\endgroup\$ – Keeta - reinstate Monica Commented Aug 27, 2019 at 18:51
- \$\begingroup\$ Plus, from a purely physical point of view, force, and thus the power needed to propel a fan faster goes up quadratically with speed. So, most definitively even a hypothetical "wasteful" fan that burns energy in a restistor turning slower would likely be more energy-efficient. \$\endgroup\$ – Damon Commented Aug 27, 2019 at 20:17
Resistors can be used to control motor speed an you described, but they must dissipate a lot of heat. The preferred speed control method is to switch the value of capacitor that is connected in series with the auxiliary winding of the single-phase motor. See Speed control for PSC induction motor As illustrated in that question, it is a natural characteristics of a fan that it requires less torque to operate at lower speeds. That mean that less power is used, since power is torque multiplied by speed. An increased proportion of that power is dissipated in the rotor of the motor, but less total power used and less power is dissipated in the rotor as compared to full-speed operation.
Shaded-pole Motors
Another answer describes much the same performance for shaded-pole motors. Shaded pole motors tend to be used for smaller fans such as exhaust fans for bathrooms and stove hoods. Window fans and pedestal fans are more likely to use the capacitor-run or permanent-split-capacitor (PSC) motor described above.
- \$\begingroup\$ I just assumed they were controlled wastefully using resistors, good to know there's more it. Thanks for your response. \$\endgroup\$ – Chorlton2080 Commented Aug 27, 2019 at 13:25
Not the answer you're looking for? Browse other questions tagged power motor ac induction-motor or ask your own question .
- The Overflow Blog
- Community Products Roadmap Update, October 2024
- Meet the AI native developers who build software through prompt engineering
- Featured on Meta
- Preventing unauthorized automated access to the network
- Upcoming initiatives on Stack Overflow and across the Stack Exchange network...
Hot Network Questions
- Making small talk: Which verbs most commonly accept “small talk” as their direct object?
- Diagonalisation in the proof of undecidability of the acceptance problem for Turing Machines
- Why not send a Mars rover to Titan?
- Were US men and women ever so divided in national polls?
- Inductive effect explanation with MO theory
- SwiftUI TimeUnits-Converter App
- Thesis part was flagged as AI generated even though it is all original
- What is the situation when a contract is altered during transmission?
- While swimming, your speed is reduced to zero. Do you start sinking?
- How much of its receive spectrum is an IC-7300 or similar radio actually sampling?
- How to assign credit on Drupal.org to a GitLab user whose name is not a link?
- Does “redeeming quality” simply mean “positive quality” today?
- Is it possible to climb to the outer side of Sigil?
- Do I need to notify the airlines that I now have Global Entry?
- Linking verbs vs negatives
- Identification: Older Sci-Fi anime involving a parallel universe where a group of elite fighters fight their alternate universe counterparts
- Can I use 给 for inanimate objects?
- Concocting a fourth spatial dimension that can support wormhole-like travel and not mess up life?
- Transformer dot convention
- Why does my iPhone 16 Pro have iOS 18.0 but I can’t find a way to activate developer mode anywhere?
- How to deal with mistakes or embarrassing moments in front of peers?
- How is AES-128 still considered to be quantum resistant?
- (Portuguese) Diacritics on Capital Letters Messing Up with Line Spacing
- Can a carbon crank be safely put into a vice?
- AMCA Connect / Members Login
Mitigating System Effect to Optimize Fan Performance and Efficiency
Date: 2024-06-04 14:52:00
By : Mike Humann, The New York Blower Company
This article appeared in the 2020 edition of AMCA inmotion magazine .
Fans used to move air in industrial and commercial applications are tested and rated in a laboratory under ideal conditions—that is, conditions designed to enable the equipment to achieve its maximum performance. As anyone who has set foot on a building site can attest, however, the conditions under which fans are put into service seldom are—and often are far from—ideal. The difference between how a fan performs installed in the field and how it performed when tested in a laboratory can be attributed to a phenomenon known as system effect.
This article will describe system effect, its causes, and its impact on fan performance. Additionally, it will discuss strategies for minimizing, eliminating, or avoiding system effect to achieve optimal, reliable performance once a fan is installed.

Credit: Mikall Grachikoy/Bigstock
System Effect and Its Impacts
System effect refers to losses in air-system performance caused by adverse flow conditions (excess turbulence or swirl) at or near the fan. System effect can occur at a fan’s inlet or outlet or both. Often, it results from changes to system design—commonly involving the length, width, and/or transition points of ductwork—made during the fan-installation process.
The only way to overcome system effect and achieve specified airflow volume is to increase fan speed. Increasing fan speed, however, results in increased energy consumption (just a 10-percent increase in fan speed will result in a 33-percent increase in energy consumption) and costs and greater stress on system components. Additionally, it may prevent the motor, electrical conduit, starter, and disconnect from achieving the necessary brake horsepower.
Figure 1 shows the impact system effect has on system performance.
In addition to hindering fan performance, system effect can increase noise and vibration and lead to premature impeller or bearing failure. The associated performance testing, engineering analysis, field service, long-term maintenance, and lost production from unplanned downtime and extended startup can be costly.
With any installation, system effect and all other system-associated losses must be considered to understand how a fan will perform relative to its laboratory tests once installed. During the fan-selection process, the combined impact of those losses should be taken into account. For optimal system performance and cost-effective operation for years to come, best practices for fan ducting and installation need to be followed.
Laboratory vs. Real-World Conditions
To recognize how system effect impacts air-system performance, it is important to understand the conditions under which fans are tested in a laboratory. Housed fans typically are tested with an open inlet that includes a bell mouth to eliminate entry losses and achieve uniform airflow across the inlet. The discharge includes a straight run of ductwork that produces fully developed airflow (free of swirl or turbulence) prior to the air entering the test chamber. Uniform, fully developed airflow enables a fan to move air efficiently and quietly through a duct system without causing excessive vibration. In the field, however, inlet and outlet conditions rarely mimic those of laboratory-test setups, as the installation and ducting are influenced by the existing infrastructure and space limitations.
Common Causes of System Effect
The most common causes of system effect include uneven or spinning airflow at a fan’s inlet, obstructions to airflow at the inlet or outlet, improperly configured ductwork at the inlet or outlet, and/or failure to correct for losses caused by fan accessories.
System effect can be avoided by accounting for all factors, including the shape of the transition points between the fan and existing ducts, ductwork configuration close to the fan, and accessories. For optimum air performance, airflow at the fan’s inlet needs to be uniform, symmetrical, and free of swirl. Similarly, airflow must be able to diffuse and fully develop across the fan’s outlet. Even minor improvements to airflow stability can reduce system effect and, in turn, increase fan performance and operating efficiency.
An inlet vane damper is a modulating device that affects fan performance. As a damper is closed, air begins to pre-spin into the fan; the fan wheel no longer can move as much air, and flow, pressure, and brake horsepower all decrease. Even when a damper is fully open, the vanes interfere with normal flow and reduce fan performance. If the losses are not accounted for, the fan will have to run at a speed higher than the one specified during fan selection. Fans should be tested with accessories that determine the losses and the fan speed required to overcome them.
Reducing System Effect at a Fan’s Inlet
A lack of uniform airflow entering a fan’s inlet is one of the greatest and most common causes of system effect. Often, these losses are the result of elbows and isolation dampers being installed too close to a fan’s inlet.
Depending on the application, a variety of strategies can be employed to improve airflow at a fan’s inlet. Take, for example, the 90-degree round elbow located at the inlet of a fan shown in Figure 2 . Air entering the fan wheel is not uniform, loading on various parts of the fan wheel instead of at the center as designed for optimal performance. Some air is circulating back into the elbow, creating additional losses. In this case, the addition of turning vanes in the elbow will help direct air toward the center of the fan wheel.
Figure 3 shows the effect of a rectangular inlet box mounted directly to the inlet of a fan. Again, the fan wheel is not being uniformly loaded, resulting in performance loss. With an inlet box, the cavity, or dead area, below the outlet is where air will get hung up, creating additional losses. Improving the shape of the inlet box or adding straightening vanes can help to redirect the flow of air into the wheel.
Figure 4 shows air entering a fan from the side as opposed to straight through the inlet. The air is spinning in the opposite direction of the fan wheel. Consequently, the fan is having to work harder, resulting in greater energy consumption and stress on fan components.
Reducing System Effect at a Fan’s Outlet
Similar to inlet flow, outlet flow is impacted significantly by the placement and distancing of ductwork and dampers. The effective length of ductwork at a fan’s outlet is one of the most important factors in fan and system efficiency. In most cases, the profile of the air coming out of a fan is asymmetrical, causing turbulence and a lack of static-pressure regain.
For symmetrical and uniform flow to be achieved, outlet ducting must be long enough to allow airflow to diffuse and fully develop. This is called 100-percent effective duct length. As a rule of thumb, the effective length of outlet ducting should be no less than 2.5 duct diameters when duct velocity is 2,500 fpm (13 m/s) or less. For every additional 1,000 fpm (5 m/s), one duct diameter should be added.
Figure 5 shows the velocity profile of air as it exits a fan. Air is forced against the outside of the scroll, resulting in uneven flow at the outlet. An effective run of ductwork allows for a uniform velocity profile. Note that at approximately 50 percent of effective duct length the fan achieves approximately 80 percent of its pressure regain.
In addition to effective duct length, the placement and direction of elbows is significant at a fan’s outlet. An elbow installed too close to the outlet will result in a significant loss of airflow. If the elbow turns in the opposite direction of the fan’s rotation, the loss will be even greater. When a design requires the installation of an elbow, a minimum of two to three duct lengths is recommended to allow the velocity profile of air exiting the fan to develop across the ductwork.
By and large, most fan-performance deficiencies are the result of improper system design. This is because fans are simple, standard mechanical devices, while systems are complex and unique, with many installation variables that can adversely impact performance.When designing a fan system for optimal operation, remember to allow enough room for needed accessories and appropriate ducting connecting the fan to the larger system. The long-term cost savings will be worth the extra effort upfront. Additionally, because fan installations are so customized, it is important to partner with a knowledgeable and experienced vendor to ensure optimum system performance, efficiency, and longevity.

Figure 1. Impact of system effect on system performance. Source: AMCA “System Effect” online educational module

Figure 2. Non-uniform airflow into a fan inlet inducated by a 90-degree, three piece section elbow- no turning vanes.

Figfure 3. Non-uniform airflow into a fan inlet induced by a rectangular inlet duct.

Figure 4. Example of a forced inlet vortex.

Figure 5. System-effect curves for outlet ducts—centrifugal fans.
About the Author
Mike Humann is manager of products and applications for The New York Blower Co. During his 10-year career, he has presented at conferences and conducted training sessions on topics including system effect, fan applications, and custom fan modifications. He has a bachelor’s degree with a concentration in physics from Elmhurst University.
Sidebar: System Effect in Effect

After a neighbor complained about the noise generated by this backward-inclined fan, which exhausted fumes from a tank at a rate of 50,000 cu ft (3,750 lb) per minute, the owner sought to redirect the noise by reversing the flow of air so that the air came back toward the fan. It was a self-defeating proposition: The air turbulence caused by the 180-degree turn at the outlet and the speed at which the fan had to run to overcome the system effect actually increased the noise.

Instead of a rectangular-to-round transition from the fan inlet, a transition plate is being used in this application, resulting in the bottom-loading of air on the fan wheel.

A fan and collector purchased at a salvage auction. The owner used a 50-gal. drum as part of the duct system between the fan and collector. In addition to a base that is unstable, there is no length of ductwork at the fan outlet to establish uniform airflow.

Pressure blowers used in a combustion-air system. The 90-degree elbows are turning air in the opposite direction it exits the fans. The system effect could have been avoided with upblast fans.

This process fan exhausting to atmosphere has to overcome system effect resulting from the use of a cutoff sheet in conjunction with a rectangular duct and a 90-degree-elbow turn without any run of ductwork.
- Energy Efficiency & System Performance
- Indoor Air Quality & Ventilation
- Occupant & Building Protection
- Large Diameter Ceiling Fans
- Path to CRP
- Product Search
- Certification Resources
- Violations & Suspended Products
- inMotion Magazine
- Meetings & Events
- Meet the People Behind AMCA
- Society Guidelines
- Become A Member
- Find Members
- Member Sign-in
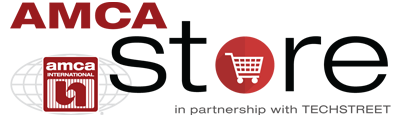
Air Movement and Control Association International, Inc.

Snapsolve any problem by taking a picture. Try it in the Numerade app?
Information
- Author Services
Initiatives
You are accessing a machine-readable page. In order to be human-readable, please install an RSS reader.
All articles published by MDPI are made immediately available worldwide under an open access license. No special permission is required to reuse all or part of the article published by MDPI, including figures and tables. For articles published under an open access Creative Common CC BY license, any part of the article may be reused without permission provided that the original article is clearly cited. For more information, please refer to https://www.mdpi.com/openaccess .
Feature papers represent the most advanced research with significant potential for high impact in the field. A Feature Paper should be a substantial original Article that involves several techniques or approaches, provides an outlook for future research directions and describes possible research applications.
Feature papers are submitted upon individual invitation or recommendation by the scientific editors and must receive positive feedback from the reviewers.
Editor’s Choice articles are based on recommendations by the scientific editors of MDPI journals from around the world. Editors select a small number of articles recently published in the journal that they believe will be particularly interesting to readers, or important in the respective research area. The aim is to provide a snapshot of some of the most exciting work published in the various research areas of the journal.
Original Submission Date Received: .
- Active Journals
- Find a Journal
- Journal Proposal
- Proceedings Series
- For Authors
- For Reviewers
- For Editors
- For Librarians
- For Publishers
- For Societies
- For Conference Organizers
- Open Access Policy
- Institutional Open Access Program
- Special Issues Guidelines
- Editorial Process
- Research and Publication Ethics
- Article Processing Charges
- Testimonials
- Preprints.org
- SciProfiles
- Encyclopedia
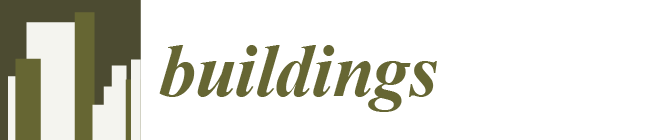
Article Menu
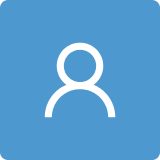
- Subscribe SciFeed
- Recommended Articles
- Google Scholar
- on Google Scholar
- Table of Contents
Find support for a specific problem in the support section of our website.
Please let us know what you think of our products and services.
Visit our dedicated information section to learn more about MDPI.
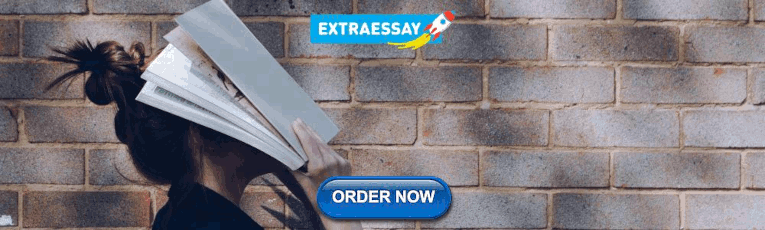
JSmol Viewer
Prediction of the impact of air speed produced by a mechanical fan and operative temperature on the thermal sensation.
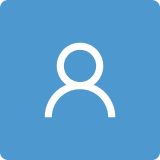
1. Introduction
Objective of this work, 2. research methods, 2.1. the calibration exercise setup (stage 1), 2.2. the parametric investigation setup (stage 2), 3.1. the calibration exercise (stage 1), 3.2. the matrices for thermal comfort obtained with parametric investigation (stage 2), 4. discussion, 5. conclusions, author contributions, acknowledgments, conflicts of interest, nomenclatures.
ASHRAE | American Society of Heating, Refrigerating and Air-Conditioning Engineers |
CE | Cooling effect (in °C) |
CFD | Computer fluid dynamics |
CSPSV | Color sequence particle streak velocimetry |
DTS | Dynamic thermal sensation |
EXP | Environmental chamber |
NV | Natural ventilation |
OPU | Percentages of unacceptability |
PMV | Predicted mean vote |
PPD | Predicted percentage of dissatisfied |
RAC | Room air conditioning |
RPM | Rotations per minute |
Skm | Mean skin temperature (in °C) |
TSV | Thermal sensation votes |
- IEA. The Future of Cooling. Opportunities for Energy-Efficient Air Conditioning. Organisation for Economic Co-operation and Development. International Energy Agency. Available online: http://www.iea.org/publications/freepublication/The_Future_of_Cooling.pdf (accessed on 22 May 2018).
- IEA. Cooling. International Energy Agency. Available online: https://www.iea.org/reports/cooling (accessed on 22 May 2018).
- CIBSE AM 10. Applications Manual 10: Natural Ventilation ; The Chartered Institution of Building Services Engineers: London, UK, 2005. [ Google Scholar ]
- de Faria, L.; Cook, M.; Loveday, D.; Angelopoulos, C.; Shukla, Y.; Rawal, R.; Manu, S.; Mishra, D.; Patel, J.; Saranya, A. Design charts to assist on the sizing of natural ventilation for cooling residential apartments in India. In Proceedings of the BS 2019: 16th IBPSA International Conference & Exhibition, Rome, Italy, 2–4 September 2019; International Building Performance Simulation Association: Wisconsin, WI, USA, 2020. [ Google Scholar ] [ CrossRef ]
- Cook, M.; Shukla, Y.; Rawal, R.; Loveday, D.; de Faria, L.C.; Angelopoulos, C. Low Energy Cooling and Ventilation for Indian Residences Design Guide ; CEPT Research and Development Foundation—CRDF: Ahmedabad, India, 2020; Volume 1, 61p, ISBN 978-1-8380310-0-8. [ Google Scholar ]
- ASHRAE/ANSI Standard-55. Thermal Environmental Conditions for Human Occupancy ; American Society of Heating and Air-Conditioning Engineers: Atlanta, GA, USA, 2017. [ Google Scholar ]
- Fanger, P.O. Thermal Comfort ; Danish Technical Press: Copenhagen, Denmark, 1970; ISBN 9780070199156. [ Google Scholar ]
- ISO 7730. Ergonomics of the Thermal Environment—Analytical Determination and Interpretation of Thermal Comfort Using Calculation of the PMV and PPD Indices and Local Thermal Comfort Criteria ; International Standard Organization: Geneva, Switzerland, 2005. [ Google Scholar ]
- Callahan, M.; Gum, H. Development of a High Efficiency Ceiling Fan. In Proceedings of the Twelfth Symposium on Improving Building Systems in Hot and Humid Climates, San Antonio, TX, USA, 15–17 May 2000; pp. 270–277. [ Google Scholar ]
- Santamouris, M. Ceiling Fans ; AIVC—Air Infiltration and Ventilation Centre: Sint-Stevens-Woluwe, Belgium, 2007; pp. 1–12. [ Google Scholar ]
- Santamouris, M. Ventilation for Comfort and Cooling: The State of the Art. In Building Ventilation: The State-of-the-Art ; Santamouris, M., Wouters, P., Eds.; Earthscan: London, UK, 2007; pp. 217–246. [ Google Scholar ] [ CrossRef ]
- Fiala, D.; Psikuta, A.; Jendritzky, G.; Paulke, S.; Nelson, D.; Van Marken Lichtenbelt, V.; Frijns, A. Physiological modeling for technical, clinical and research applications. Front. Biosci. 2010 , 1 , 939–968. [ Google Scholar ] [ CrossRef ] [ PubMed ] [ Green Version ]
- Scheatzle, D.G.; Wu, H.; Yellott, J. Extending the summer comfort envelope with ceiling fans in hot arid climates. ASHRAE Trans. 1989 , 95 , 269–280. [ Google Scholar ]
- Mallic, F.H. Thermal comfort and building design in the tropical climates. Energy Build. 1996 , 23 , 161–167. [ Google Scholar ] [ CrossRef ]
- de Dear, R.; Brager, G.S.; Cooper, D. Developing an adaptive model of thermal comfort and preference. In ASHRAE RP-884 Final Report ; American Society of Heating and Air-Conditioning Engineers: Atlanta, GA, USA, 1997; Available online: https://escholarship.org/uc/item/4qq2p9c6 (accessed on 18 September 2020).
- de Dear, R.; Kim, J.; Parkinson, T. Residential Adaptive Comfort in a Humid Subtropical Climate—Sydney Australia. Energy Build. 2018 , 158 , 1296–1305. [ Google Scholar ] [ CrossRef ]
- de Dear, R.; Brager, G.S. Thermal comfort in naturally ventilated buildings: Revisions to ASHRAE Standard 55. Energy Build. 2002 , 34 , 549–561. [ Google Scholar ] [ CrossRef ] [ Green Version ]
- Chandra, S.; Fairey, P.; Houston, M. Cooling with Ventilation ; Solar Energy Research Institute, U.S. Department of Energy: Cocoa, FL, USA, 1986.
- Mcintyre, D. Preferred air speed for comfort in warm conditions. ASHRAE Trans. 1978 , 84 , 264–277. [ Google Scholar ]
- Rohles, F.; Konz, S.; Jones, B. Ceiling fans as extenders of the summer comfort envelope. ASHRAE Trans. 1983 , 89 , 245–263. [ Google Scholar ]
- Indraganti, M. Behavioural adaptation and the use of environmental controls in summer for thermal comfort in apartments in India. Energy Build. 2010 , 42 , 1019–1025. [ Google Scholar ] [ CrossRef ]
- Indraganti, M. Adaptive use of natural ventilation for thermal comfort in Indian apartments. Build. Environ. 2010 , 45 , 1490–1507. [ Google Scholar ] [ CrossRef ]
- Indraganti, M.; Ooka, R.; Rijal, H. Significance of air movement for thermal comfort in warm climates: A discussion in Indian context. In Proceedings of the 7th Windsor Conference: The Changing Context of Comfort in an Unpredictable World, Cumberland Lodge, Windsor, UK, 12–15 April 2012. [ Google Scholar ]
- Gao, Y.; Zhang, H.; Arens, E.; Present, E.; Ning, B.; Zhai, Y.; Pantelic, J.; Luo, M.; Zhao, L.; Raftery, P.; et al. Ceiling fan air speeds around desks and office partitions. Build. Environ. 2017 , 124 , 412–440. [ Google Scholar ] [ CrossRef ] [ Green Version ]
- Liu, S.; Lipczynska, A.; Schiavon, S.; Arens, E. Detailed experimental investigation of air speed field induced by ceiling fans. Build. Environ. 2018 , 142 , 342–360. [ Google Scholar ] [ CrossRef ] [ Green Version ]
- Raftery, P.; Douglass-James, D. Ceiling Fan Design Guide ; University of California Berkeley, The Centre for the Built Environment: Berkeley, CA, USA, 2020; Available online: https://escholarship.org/uc/item/6s44510d (accessed on 21 June 2021).
- Oh, W.; Kato, S. The effect of airspeed and wind direction on human’s thermal conditions and air distribution around the body. Build. Environ. 2018 , 141 , 103–116. [ Google Scholar ] [ CrossRef ]
- Raftery, P.; Fizer, J.; Chen, W.; He, Y.; Zhang, H.; Arens, E.; Schiavon, S.; Paliaga, G. Ceiling fans: Predicting indoor air speeds based on full scale laboratory measurements. Build. Environ. 2019 , 155 , 210–223. [ Google Scholar ] [ CrossRef ] [ Green Version ]
- Falahat, A. Optimization of Flow Coefficient in Tubeaxial Fan at a Different Hub to Tip Ratio. Int. Rev. Mech. Eng. 2011 , 5 , 1095–1101. [ Google Scholar ]
- Adeeb, E.; Maqsood, A.; Mushtaq, A. Effect of Number of Blades on Performance of Ceiling Fans. In MATEC Web of Conferences ; EDP Sciences: Les Ulis, France, 2015; Volume 28, Available online: http://www.matec-conferences.org/10.1051/matecconf/20152802002 (accessed on 15 January 2018).
- Schmidt, K.; Patterson, D. Performance Results for a High Efficiency Tropical Ceiling Fan and Comparisons with Conventional Fans Demand Side Management via Small Appliance Efficiency. Renew. Energy 2001 , 22 , 169–176. Available online: www.elsevier.com/locate/renene (accessed on 22 January 2018). [ CrossRef ]
- Jain, A.; Upadhyay, R.R.; Chandra, S.; Saini, M.; Kale, S. Experimental investigation of the flow field of a ceiling fan. In Proceedings of the ASME 2004 Heat Transfer/Fluids Engineering Summer Conference, Charlotte, NC, USA, 11–15 July 2004; American Society of Mechanical Engineers. pp. 93–99. [ Google Scholar ]
- Wang, H.; Zhang, H.; Hu, X.; Luo, M.; Wang, G.; Li, X.; Zhu, Y. Measurement of airflow pattern induced by ceiling fan with quad-view colour sequence particle streak velocimetry. Build. Environ. 2019 , 152 , 122–134. [ Google Scholar ] [ CrossRef ] [ Green Version ]
- Ge, H.W.; Norconk, M.; Lee, S.Y.; Naber, J.; Wooldridge, S.; Yi, J. PIV measurement and numerical simulation of fan-driven flow in a constant volume combustion vessel. Appl. Therm. Eng. 2014 , 64 , 19–31. [ Google Scholar ] [ CrossRef ]
- Babich, F.; Cook, M.; Loveday, D.; Cropper, P. Numerical modelling of thermal comfort in non-uniform environments using real-time coupled simulation models. In Proceedings of the Building Simulation and Optimisation 2016: 3rd IBPSA-England Conference, BPSA, Newcastle upon Tyne, UK, 12–14 September 2016; pp. 4–11. [ Google Scholar ]
- Babich, F.; Cook, M.; Loveday, D.; Rawal, R.; Shukla, Y. Transient three-dimensional CFD modelling of ceiling fans. Build. Environ. 2017 , 123 , 37–49. [ Google Scholar ] [ CrossRef ] [ Green Version ]
- Li, J.; Hou, Y.; Liu, J.; Wang, Z.; Li, F. Window purifying ventilator using a cross-flow fan: Simulation and optimization. Build. Simul. 2016 , 9 , 481–488. [ Google Scholar ] [ CrossRef ]
- Chen, Q.; Liu, S.; Gao, Y.; Zhang, H.; Arens, E.; Zhao, L.; Liu, J. Experimental and numerical investigations of indoor air movement distribution with an office ceiling fan. Build. Environ. 2018 , 130 , 14–26. [ Google Scholar ] [ CrossRef ] [ Green Version ]
- Procel. Ventiladores de Teto. Selo Procel: Programa Nacional de Conservação de Energia Elétrica. Centro Brasileiro de Informação de Eficiência Energética. Eletrobrás. 06/08/2019. Available online: http://www.procelinfo.com.br/ (accessed on 22 October 2019).
- DOE. Improving Fan System Performance: A Sourcebook for Industry ; The U.S. Department of Energy Efficiency and Renewable Energy (EERE), the Department of Energy’s (DOE) Industrial Technologies Program and the Air Movement and Control Association (AMCA): Washington, DC, USA, 2003; 92p. Available online: https://www.nrel.gov/docs/fy03osti/29166.pdf (accessed on 3 September 2020).
- Fiala, D.; Lomas, K.; Stohrer, M. Dynamic Simulation of Human Heat Transfer and Thermal Comfort. Ph.D. Thesis, De Montfort University, Leicester, UK, June 1998. Available online: http://hdl.handle.net/2086/4129 (accessed on 28 December 2020).
- Fiala, D.; Lomas, K.; Stohrer, M. Computer prediction of human thermoregulatory and temperature responses to a wide range of environmental conditions. Int. J. Biometeorol. 2001 , 45 , 143–159. [ Google Scholar ] [ CrossRef ] [ PubMed ]
- Fiala, D.; Lomas, K.; Stohrer, M. First Principles Modeling of Thermal Sensation Responses in Steady-State and Transient Conditions. ASHRAE Trans. 2003 , 109 , 179–186. [ Google Scholar ]
- Cropper, P.; Yang, T.; Cook, M.; Fiala, D.; Yousaf, R. Coupling a model of human thermoregulation with computational fluid dynamics for predicting human-environment interaction. J. Build. Perform. Simul. 2010 , 3 , 233–243. [ Google Scholar ] [ CrossRef ] [ Green Version ]
- de Faria, L.C.; Romero, M.; Pirró, L. Evaluation of a Coupled Model to Predict the Impact of Adaptive Behaviour in the Thermal Sensation of Occupants of Naturally Ventilated Buildings in Warm-Humid Regions. Special Issue ‘Indoor Environment in Sustainable Buildings’. Sustainability 2020 , 13 , 255. [ Google Scholar ] [ CrossRef ]
- Lamberts, R.; Andreasi, W.A. Thermal Comfort in Buildings Located in Regions of Hot and Humid Climate of Brazil. Laboratory of Energy Efficiency in Buildings, LABEEE. UFSC. 2009. Available online: https://www.researchgate.net/publication/242253258_Thermal_comfort_in_buildings_located_in_regions_of_hot_and_humid_climate_of_Brazil/references (accessed on 10 May 2020).
- Andreasi, W.A.; Lamberts, R.; Cândido, C. Thermal acceptability assessment in buildings located in hot and humid regions in Brazil. Build. Environ. 2010 , 45 , 1225–1232. [ Google Scholar ] [ CrossRef ]
- de Dear, R. Recent Enhancements to the Adaptive Comfort Standard in ASHRAE 55-2010. In Proceedings of the 45th Annual Conference of the Architectural Science Association, ANZAScA 2011, Sydney, Australia, 14–16 November 2011. [ Google Scholar ]
- ANSYS. ANSYS ICEM CFD R16.0. ANSYS, Inc., 2016. Available online: https://www.ansys.com/training-center/course-catalog/fluids/introduction-to-ansys-icem-cfd (accessed on 19 January 2022).
- ANSYS. ANSYS CFX R19.1. ANSYS, Inc., 2018. Available online: https://www.ansys.com/products/fluids/ansys-cfx/ (accessed on 19 January 2022).
- Celik, L.; Ghia, U.; Roache, P.; Freitas, C.; Coleman, H.; Raad, P. Procedure for estimation and reporting of uncertainty due to discretization in CFD applications. J. Fluids Eng. Trans. 2008 , 130 , 0780011–0780014. [ Google Scholar ] [ CrossRef ] [ Green Version ]
- Hajdukiewicz, M.; Geron, M.; Keane, M. Formal calibration methodology for CFD models of naturally ventilated indoor environments. Build. Environ. 2013 , 59 , 290–302. [ Google Scholar ] [ CrossRef ] [ Green Version ]
- Naghshpour, S. Statistics for Economics ; Business Expert Press: New York, NY, USA, 2012; p. 475. [ Google Scholar ]
- Croft, A.; Davison, R. Foundation Maths , 5th ed.; Pearson: Harlow, UK, 2010. [ Google Scholar ]
- Tartarini, F.; Schiavon, S.; Cheung, T.; Hoyt, T. CBE Thermal Comfort Tool: Online tool for thermal comfort calculations and visualizations. SoftwareX 2020 , 12 , 100563. [ Google Scholar ] [ CrossRef ]
- CBE. Thermal Comfort Tool. Available online: https://comfort.cbe.berkeley.edu/ (accessed on 21 July 2021).
- Cheung, T.; Schiavon, S.; Parkinson, T.; Li, P.; Brager, G. Analysis of the accuracy on PMV—PPD model using the ASHRAE Global Thermal Comfort Database II. Build. Environ. 2019 , 153 , 205–217. [ Google Scholar ] [ CrossRef ] [ Green Version ]
Click here to enlarge figure
Air Speed (m/s) | Corresponding Rise in the Operative Temperature (°C) | Upper Limit for the Operative Temperature (°C) |
---|---|---|
0.1 | - | 27.00 |
0.6 | 2.80 | 29.80 |
0.9 | 3.40 | 30.40 |
1.2 | 3.75 | 30.75 |
1.5 | 4.00 | 31.00 |
Velocity | Momentum Sources for the Cylindrical Components (kg/m s ) | ||
---|---|---|---|
Modes | Axial | Radial | Theta |
Velocity VI | 55.00 | 0.0 | 8.00 |
Velocity V | 38.50 | 0.0 | 5.60 |
Velocity IV | 27.50 | 0.0 | 4.00 |
Velocity III | 13.75 | 0.0 | 2.00 |
Velocity II | 5.50 | 0.0 | 0.80 |
Velocity I | 2.75 | 0.0 | 0.40 |
Velocity Mode | RPM | Momentum Sources for the Cylindrical Components | Air Speed at 1.0 m | Flow Rate | ||
---|---|---|---|---|---|---|
Axial (kg/m s ) | Radial (kg/m s ) | Theta (kg/m s ) | Above Floor (m/s) | (m /s) | ||
Velocity VI | 330 | 55.00 | 0.0 | 8.00 | 2.75 | 3.93 |
Velocity V | 260 | 38.50 | 0.0 | 5.60 | 2.40 | 3.09 |
Velocity IV | 210 | 27.50 | 0.0 | 4.00 | 2.11 | 2.50 |
Velocity III | 130 | 13.75 | 0.0 | 2.00 | 1.53 | 1.55 |
Velocity II | 95 | 5.50 | 0.0 | 0.80 | 0.95 | 1.13 |
Velocity I | 42 | 2.75 | 0.0 | 0.40 | 0.59 | 0.50 |
MDPI stays neutral with regard to jurisdictional claims in published maps and institutional affiliations. |
Share and Cite
Faria, L.C.d.; Romero, M.d.A.; Porras-Amores, C.; Pirró, L.F.d.S.; Saez, P.V. Prediction of the Impact of Air Speed Produced by a Mechanical Fan and Operative Temperature on the Thermal Sensation. Buildings 2022 , 12 , 101. https://doi.org/10.3390/buildings12020101
Faria LCd, Romero MdA, Porras-Amores C, Pirró LFdS, Saez PV. Prediction of the Impact of Air Speed Produced by a Mechanical Fan and Operative Temperature on the Thermal Sensation. Buildings . 2022; 12(2):101. https://doi.org/10.3390/buildings12020101
Faria, Luciano Caruggi de, Marcelo de Andrade Romero, César Porras-Amores, Lucia Fernanda de Souza Pirró, and Paola Villoria Saez. 2022. "Prediction of the Impact of Air Speed Produced by a Mechanical Fan and Operative Temperature on the Thermal Sensation" Buildings 12, no. 2: 101. https://doi.org/10.3390/buildings12020101
Article Metrics
Article access statistics, further information, mdpi initiatives, follow mdpi.
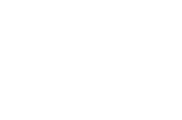
Subscribe to receive issue release notifications and newsletters from MDPI journals
Is A Low Or High AC Fan Speed Better? (Explained & Solved!)
Most of today’s air conditioning systems offer a fan that can be operated at both a high and low speed.
Find out which is better for specific scenarios and why.
Here’s if a Low or High AC Fan Speed Is Better:
A fan operating at the highest speed possible will without a doubt produce the most airflow in the least amount of time. This, of course, will also come at the cost of noise. Despite that, this doesn’t necessarily mean high speed is the best setting for all situations. Take a very hot, humid day for example. A low setting could be a much more effective option. Why? Simply because it will run longer, removing unwanted moisture while it is running.
Does Fan Speed Affect AC?
Yes, you better believe that fan speed will affect the AC. The fan is an integral component of any HVAC system. How it operates will greatly affect the outcome of the AC.
To start, it is important to realize that a residential or commercial air conditioning system contains two fans.
They would be the outdoor fan (condenser fan) or the indoor fan (air handler fan).
The purpose of the outdoor fan is to remove hot air from the condenser and compressor so that the refrigerant in the copper lines can change from vapor to liquid.
When most homeowners or average Joes refer to an air conditioner fan or an AC fan, they are not referring to the condenser fan. In fact, this is one of the more overlooked components.
When most average individuals refer to an air conditioner fan, they are talking about the indoor fan or the air handler fan.
This is the more noticeable of the two components, as this fan’s responsibility is to force air through the ducts and into the home.
When you stand over or under your vents in the home or office, it’s the indoor fan that moves the air. This is the fan most people refer to simply because it is the one that most affects them.
While the outdoor fan does play a huge role in the overall operation of the AC system, it usually goes unnoticed.
With that established, the discussion about fan speeds can get underway. Most people would automatically assume that the better speed is the highest speed.
This assumption is made by many because of the physical connection. It’s the more noticeable of the two.
Now, imagine two box fans, one blowing on high and one blowing on low. What are you going to notice standing in front of the two?
You’ll notice that the high-blowing fan is forcing out more air at a higher velocity. Just because this is the case it doesn’t necessarily mean the high fan setting is always the optimal option.
Things can, unfortunately, get a bit tricky from this point. To fully grasp the concept, you need industry insight explaining how the fan also controls humidity .
The simplest way to think about it is by understanding that an air conditioner’s job is not just to specifically satisfy a temperature setting on the thermostat.
When operating properly and efficiently, the indoor air conditioning fan should also remove humidity. Think about setting your thermostat to 75 degrees F in the summer.

When the temperature in the home rises to 76 degrees F the system will startup. Imagine one system running on the high setting compared to the same one running on the low setting.
The higher setting is going to bring the temperature back down to 75 degrees F faster, causing the thermostat to satisfy and shut off.
This is where most would assume that the high setting is more efficient because it forces the thermostat to reach 75 degrees F faster. And this is true in certain respects.
However, if the fan shuts down too fast it might not remove all the humidity in the home to make it feel comfortable.
If this is the case, it’s going to cause the homeowner to visit the thermostat and turn down the temperature even further.
When the fan runs at a slower speed it’ll run longer, removing more humidity from the air.
This might take a little longer to satisfy the stat, but the process will force the system to remove more humidity during operation.
Since humidity is best described as the degrees to which the air feels, you’re always going to want to remove as much of it as possible.
If properly sized and functioning properly, your indoor fan will already be set to the most efficient settings possible.
If you are trying to tweak your fan to satisfy the thermostat or make it more comfortable in the home something is wrong.
There could be something physically wrong with the fan motor or its squirrel cage. This could also be a blockage of air in the duct system somewhere or the unit itself.
Calculating and measuring airflow can be extremely technical and difficult. Therefore, it is best to work with a professional to make sure your unit is functioning properly.
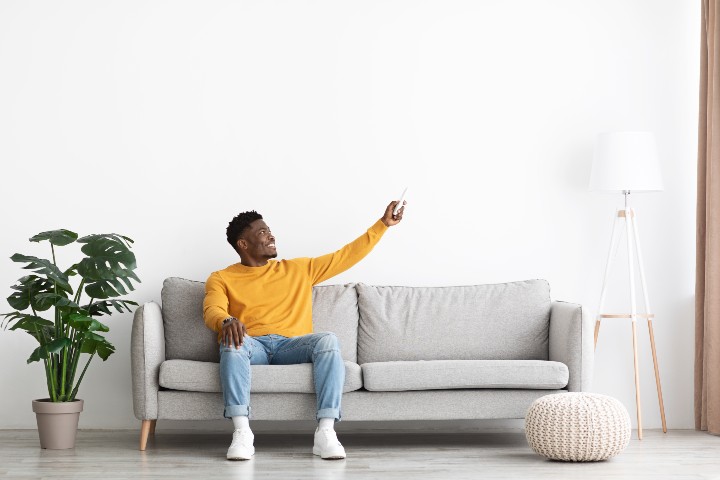
Does Higher Fan Speed Affect Electricity Consumption?
If you think fan airflow is complex, you’ve yet to touch the surface. Although there are some simple aspects to wiring, electrical currents and circuits are demandingly complicated.
According to industry experts, 90 percent of air conditioner breakdowns are due to wiring issues.
Add to that the fact that just about everything in an air conditioner runs on electricity, and you can imagine just how hard it will be to trace these circuits.
This is not to even mention the fact that electricity is extremely dangerous. Electrical shorts can cause fires and it only takes a single amp traveling across the heart to make it stop.
Most basic AC systems produce anywhere from 30 to 40 amps. To muddy things even further, a fan operating on high doesn’t consume more electricity than a low running fan.
They consume the same amount of energy. The reason for this is extremely complicated and technical.
You’ll feel like you need an electrical engineering background just to understand the theory.
However, the easiest way to think about it is in terms of voltage drop, not voltage being applied.
When you change your fan settings, you are changing the voltage drop rather than the voltage applied.
AC Designs Priority Energy
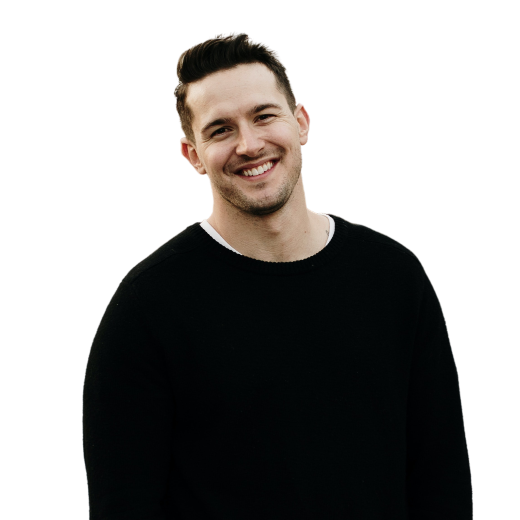
Formulating a Hypothesis Write a hypothesis about the effect of the fan spood on the acceleration of the cart Use the "if __ then __ because __ format and be sure to answer the lesson question: "How does an object's position and velocity change as the object accelorates? square
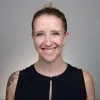
Explanation

All Subjects Homework Helper
Air pollution mitigation and CO 2 emission reduction effects of heterogeneous enterprises synergistic agglomeration
- Published: 07 October 2024
Cite this article
- Jiamin Liu 1 ,
- Xiaoyu Ma 1 , 2 ,
- Jiaoning Zhang 1 ,
- Chiqun Hu 3 &
- Qiuqiu Guo 1
In the context of synergistic pollution control, it is of great significance to explore the mitigation path of air pollution (Aip) and CO 2 emission (CO 2 ) through the heterogeneous enterprises synergistic agglomeration (Msa) represented by synergistic agglomeration of manufacturing enterprises and productive service enterprises. Based on panel data of 284 prefecture-level and above cities from 2010 to 2021, the effect of Msa on Aip and CO 2 is examined. The results indicate that the Msa curbs Aip and CO 2 . For every one unit increase in Msa, Aip decreases by 0.786 units, and CO 2 decreases by 0.122 units. This impact is effective in first–second-tier cities, central cities, and non-resource-based cities. Innovative talent mobility and green technology innovation are pathways for Msa to reduce Aip and CO 2 . In addition, as the market potential increases, the effect of Msa on Aip and CO 2 shows a leaping feature: significant promotion → significant inhibition → no impact. Environmental protection policy significantly decreases the effect of Msa inhibiting Aip and CO 2 . This study provides theoretical support for local governments to guide the synergistic agglomeration of manufacturing enterprises and productive service enterprises, and control air pollution and greenhouse gas emissions. This is a better choice to achieve co-benefit of economic and environmental welfare.
Graphical abstract
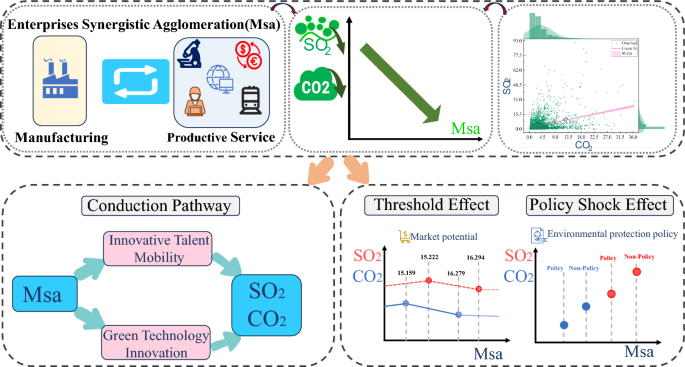
This is a preview of subscription content, log in via an institution to check access.
Access this article
Subscribe and save.
- Get 10 units per month
- Download Article/Chapter or eBook
- 1 Unit = 1 Article or 1 Chapter
- Cancel anytime
Price includes VAT (Russian Federation)
Instant access to the full article PDF.
Rent this article via DeepDyve
Institutional subscriptions
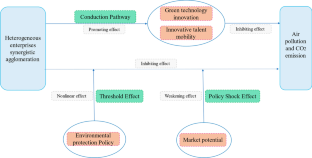
Data source: https://www.mee.gov.cn/hjzl/sthjzk/zghjzkgb/202305/P020230529570623593284.pdf .
Data source: http://www.rmzxb.com.cn/c/2022-12-27/3264252.shtml .
Data source: https://www.mee.gov.cn/hjzl/sthjzk/sthjtjnb/202301/W020230118392178258531.pdf .
19 industries include agriculture, forestry, animal husbandry, and fisheries; Mining; Manufacturing; Electricity, heat, gas and water production and supply; Construction; Wholesale and retail; Transportation, storage and postal services; Accommodation and catering; Information transmission, software and information technology services; Finance; Real estate; Leasing and business services; Scientific research and technology services; Water conservancy, environment and public facilities management; Residential services; Repair and other services; Education industry; Health and social work; Culture, sports and entertainment; Public management, social security, and social organization industry.
Data source: https://www.ipe.org.cn .
Apergis, N., & Salim, R. (2015). Renewable energy consumption and unemployment: Evidence from a sample of 80 countries and nonlinear estimates. Applied Economics, 47 (52), 5614–5633. https://doi.org/10.1080/00036846.2015.1054071
Article Google Scholar
Bai, T., Qi, Y., Li, Z., & Xu, D. (2023). Digital economy, industrial transformation and upgrading, and spatial transfer of carbon emissions: The paths for low-carbon transformation of Chinese cities. Journal of Environmental Management, 344 , 118528. https://doi.org/10.1016/j.jenvman.2023.118528
Article CAS Google Scholar
Bernard, A. B., Dhyne, E., Magerman, G., Manova, K., & Moxnes, A. (2022). The origins of firm heterogeneity: A production network approach. Journal of Political Economy, 130 (7), 1765–1804. https://doi.org/10.1086/719759
Bernardo, G., & D’Alessandro, S. (2016). Systems-dynamic analysis of employment and inequality impacts of low-carbon investments. Environmental Innovation and Societal Transitions, 21 , 123–144. https://doi.org/10.1016/j.eist.2016.04.006
Bollen, K. A. (2012). Instrumental variables in sociology and the social sciences. Annual Review of Sociology, 38 , 37–72. https://doi.org/10.1146/annurev-soc-081309-150141
Boyce, J. K. (1994). Inequality as a cause of environmental degradation. Ecological Economics, 11 (3), 169–178. https://doi.org/10.1016/0921-8009(94)90198-8
Cai, B., Cui, C., Zhang, D., Cao, L., Wu, P., Pang, L., Zhang, J., & Dai, C. (2019). China city-level greenhouse gas emissions inventory in 2015 and uncertainty analysis. Applied Energy, 253 , 113579. https://doi.org/10.1016/j.apenergy.2019.113579
Cai, M., Shi, Y., Ren, C., Yoshida, T., Yamagata, Y., Ding, C., & Zhou, N. (2021). The need for urban form data in spatial modeling of urban carbon emissions in China: A critical review. Journal of Cleaner Production, 319 , 128792. https://doi.org/10.1016/j.jclepro.2021.128792
Chen, F., Wang, M., & Pu, Z. (2022). The impact of technological innovation on air pollution: Firm-level evidence from China. Technological Forecasting and Social Change, 177 , 121521.
Chen, J., Shi, X., Gu, L., Wu, G., Su, T., Wang, H. M., Kim, J.-S., Zhang, L., & Xiong, L. (2023a). Impacts of climate warming on global floods and their implication to current flood defense standards. Journal of Hydrology, 618 , 129236. https://doi.org/10.1016/j.jhydrol.2023.129236
Chen, S., Tan, Z., Mu, S., Wang, J., Chen, Y., & He, X. (2023b). Synergy level of pollution and carbon reduction in the Yangtze River Economic Belt: Spatial–temporal evolution characteristics and driving factors. Sustainable Cities and Society, 98 , 104859. https://doi.org/10.1016/j.scs.2023.104859
Cheng, Y., Awan, U., Ahmad, S., & Tan, Z. (2021). How do technological innovation and fiscal decentralization affect the environment? A story of the fourth industrial revolution and sustainable growth. Technological Forecasting and Social Change, 162 , 120398. https://doi.org/10.1016/j.techfore.2020.120398
Cheng, Z. (2016). The spatial correlation and interaction between manufacturing agglomeration and environmental pollution. Ecological Indicators, 61 , 1024–1032. https://doi.org/10.1016/j.ecolind.2015.10.060
Chien, F. (2022). How renewable energy and non-renewable energy affect environmental excellence in N-11 economies? Renewable Energy, 196 , 526–534. https://doi.org/10.1016/j.renene.2022.07.013
Cong, J., Liu, X., & Zhao, X. (2014). Demarcation problems and the corresponding measurement methods of the urban carbon accounting. China Population, Resources and Environment, 24 (4), 19–26. (In Chineses).
Google Scholar
De Faria, P., Lima, F., & Santos, R. (2010). Cooperation in innovation activities: The importance of partners. Research Policy, 39 (8), 1082–1092. https://doi.org/10.1016/j.respol.2010.05.003
Descals, A., Verger, A., Yin, G., Filella, I., Fu, Y. H., Piao, S., Janssens, I. A., & Peñuelas, J. (2023). Radiation-constrained boundaries cause nonuniform responses of the carbon uptake phenology to climatic warming in the Northern Hemisphere. Global Change Bio, 29 (3), 719–730. https://doi.org/10.1111/gcb.16502
Du, M., & Zhang, Y. J. (2023). The impact of producer services agglomeration on green economic development: Evidence from 278 Chinese cities. Energy Economics . https://doi.org/10.1016/j.eneco.2023.106769
Ellison, G., Glaeser, E. L., & Kerr, W. R. (2010). What causes industry agglomeration? Evidence from coagglomeration patterns. Am Econc Rev, 100 (3), 1195–1213. https://doi.org/10.1257/aer.100.3.1195
Eswaran, M., & Kotwal, A. (2002). The role of the service sector in the process of industrialization. Journal of Development Economics, 68 (2), 401–420. https://doi.org/10.1016/S0304-3878(02)00019-6
Fan, W., Wang, F., Liu, J., Yan, B., Chen, T., Liu, S., & Zhang, H. (2023). Environmental effects of financial agglomeration under dual correlations of industry and space: Evidence from 286 prefecture-level cities in China. Environmental Impact Assessments, 98 , 106978. https://doi.org/10.1016/j.eiar.2022.106978
Feng, T., Chen, X., Ma, J., Sun, Y., Du, H., Yao, Y., Chen, Z., Wang, S., & Mi, Z. (2023). Air pollution control or economic development? Empirical evidence from enterprises with production restrictions. Journal of Environmental Management, 336 , 117611. https://doi.org/10.1016/j.jenvman.2023.117611
Fisher-Vanden, K. (2003). Management structure and technology diffusion in Chinese state-owned enterprises. Energ Policy, 31 (3), 247–257. https://doi.org/10.1016/S0301-4215(02)00033-2
Francois, J., & Hoekman, B. (2010). Services trade and policy. Journal of Economic Literature, 48 (3), 642–692. https://doi.org/10.1257/jel.48.3.642
Glaeser, E. L., & Kahn, M. E. (2010). The greenness of cities: Carbon dioxide emissions and urban development. Journal of Urban Economics, 67 (3), 404–418. https://doi.org/10.1016/j.jue.2009.11.006
Granados, J. A. T., & Spash, C. L. (2019). Policies to reduce CO 2 emissions: Fallacies and evidence from the United States and California. Environmental Science & Policy, 94 , 262–266. https://doi.org/10.1016/j.envsci.2019.01.007
Gray, W. B., & Shadbegian, R. J. (1998). Environmental regulation, investment timing, and technology choice. The Journal of Industrial Economics, 46 (2), 235–256. https://doi.org/10.1111/1467-6451.00070
Guan, J., & Yam, R. C. (2015). Effects of government financial incentives on firms’ innovation performance in China: Evidences from Beijing in the 1990s. Research Policy, 44 (1), 273–282. https://doi.org/10.1016/j.respol.2014.09.001
Guo, Q., Wang, Y., Zhang, Y., Yi, M., & Zhang, T. (2022). Environmental migration effects of air pollution: Micro-level evidence from China. Environmental Pollution, 292 , 118263. https://doi.org/10.1016/j.envpol.2021.118263
Habiba, U. M. M. E., Xinbang, C., & Anwar, A. (2022). Do green technology innovations, financial development, and renewable energy use help to curb carbon emissions? Renewable Energy, 193 , 1082–1093. https://doi.org/10.1016/j.renene.2022.05.084
Han, J., Li, G., Shen, Z., Song, M., & Zhao, X. (2022). Manufacturing transfer and environmental efficiency: Evidence from the spatial agglomeration of manufacturing in China. Journal of Environmental Management, 314 , 115039. https://doi.org/10.1016/j.jenvman.2022.115039
Harleman, M., Harris, L., Willis, M. D., Ritz, B., Hystad, P., & Hill, E. L. (2023). Changes in traffic congestion and air pollution due to major roadway infrastructure improvements in Texas. Science of the Total Environment, 898 , 165463. https://doi.org/10.1016/j.scitotenv.2023.165463
Harris, C. D. (1954). The market as a factor in the localization of industry in the United States. Annals of the Association of American Geographers, 44 (4), 315–348. https://doi.org/10.1080/00045605409352140
Hassen, S., Gebrehiwot, T., & Arega, T. (2018). Determinants of enterprises use of energy efficient technologies: Evidence from urban Ethiopia. Energ Policy, 119 , 388–395. https://doi.org/10.1016/j.enpol.2018.04.057
He, W., Zhang, H., Chen, X., & Yan, J. (2019). An empirical study about population density, economic agglomeration and carbon emission state of Chinese Provinces: based on the perspective of agglomeration economy effects, congestion effects and spatial effects. Nankai Economic Studies, 2 , 207–225. https://doi.org/10.14116/j.nkes.2019.02.011
Head, K., & Mayer, T. (2004). Market potential and the location of Japanese investment in the European Union. Review of Economics and Statistics, 86 (4), 959–972. https://doi.org/10.1162/0034653043125257
Huang, X., & Tian, P. (2023). Polluting the neighbor or benefiting thy neighbor: Effects of the clean energy development on haze pollution in China. Energy, 268 , 126685. https://doi.org/10.1016/j.energy.2023.126685
Huang, Y., & Wang, Y. (2020). How does high-speed railway affect green innovation efficiency? A perspective of innovation factor mobility. Journal of Cleaner Production, 265 , 121623. https://doi.org/10.1016/j.jclepro.2020.121623
IEA. (2021). An energy sector roadmap to carbon neutrality in China. International Energy Agency. https://www.iea.org/reports/an-energy-sector-roadmap-to-carbon-neutrality-in-china
Jing, Q., Bai, H., Luo, W., Cai, B., & Xu, H. (2018). A top-bottom method for city-scale energy-related CO 2 emissions estimation: A case study of 41 Chinese cities. Journal of Cleaner Production, 202 , 444–455. https://doi.org/10.1016/j.jclepro.2018.08.179
Ju, K., Lu, L., Chen, T., Duan, Z., Chen, D., Liao, W., Zhou, Q., Xu, Z., & Wang, W. (2022). Does long-term exposure to air pollution impair physical and mental health in the middle-aged and older adults?—A causal empirical analysis based on a longitudinal nationwide cohort in China. Science of the Total Environment, 827 , 154312. https://doi.org/10.1016/j.scitotenv.2022.154312
Karaomerioglu, D. C., & Carlaaon, B. (1999). Manufacturing in decline? A matter of definition. Economics of Innovation and New Technology, 8 (3), 175–196. https://doi.org/10.1080/10438599900000008
Karlsson, M., Alfredsson, E., & Westling, N. (2020). Climate policy co-benefits: A review. Clim Policy, 20 (3), 292–316. https://doi.org/10.1080/14693062.2020.1724070
Ke, S., He, M., & Yuan, C. (2014). Synergy and co-agglomeration of producer services and manufacturing: A panel data analysis of Chinese cities. Regional Studies, 48 (11), 1829–1841. https://doi.org/10.1080/00343404.2012.756580
Kim, Y. R., Williams, A. M., Park, S., & Chen, J. L. (2021). Spatial spillovers of agglomeration economies and productivity in the tourism industry: The case of the UK. Tourism Manage, 82 , 104201. https://doi.org/10.1016/j.tourman.2020.104201
Krugman, P., & Venables, A. J. (1995). Globalization and the inequality of nations. The Quarterly Journal of Economics, 110 (4), 857–880. https://doi.org/10.2307/2946642
Lanaspa, L., Sanz-Gracia, F., & Vera-Cabello, M. (2016). The (strong) interdependence between intermediate producer services’ attributes and manufacturing location. Economic Modelling, 57 , 1–12. https://doi.org/10.1016/j.econmod.2016.04.010
Lee, C. C., Wang, C. W., Ho, S. J., & Wu, T. P. (2021). The impact of natural disaster on energy consumption: International evidence. Energy Economics, 97 , 105021. https://doi.org/10.1016/j.eneco.2020.105021
Li, G., Xue, Q., & Qin, J. (2022a). Environmental information disclosure and green technology innovation: Empirical evidence from China. Technological Forecasting and Social Change, 176 , 121453. https://doi.org/10.1016/j.techfore.2021.121453
Li, W., Ji, Z., & Dong, F. (2022b). Spatio-temporal evolution relationships between provincial CO2 emissions and driving factors using geographically and temporally weighted regression model. Sustainable Cities and Society, 81 , 103836. https://doi.org/10.1016/j.scs.2022.103836
Li, X., Wang, Y., Zhou, H., & Shi, L. (2020). Has China’s war on pollution reduced employment? Quasi-experimental evidence from the clean air action. The Journal of Environmental Management, 260 , 109851. https://doi.org/10.1016/j.jenvman.2019.109851
Li, X., Xu, Y., & Yao, X. (2021). Effects of industrial agglomeration on haze pollution: A Chinese city-level study. Energy Policy, 148 , 111928. https://doi.org/10.1016/j.enpol.2020.111928
Liu, J., Ma, X., Jia, W., & Zhang, S. (2022). Can new-type urbanization construction narrow the urban-rural income gap? Evidence from China. Sustainability, 14 (22), 14725. https://doi.org/10.3390/su142214725
Liu, J., Ma, X., Zhao, B., & Liang, J. (2024). Does synergistic agglomeration of heterogeneous enterprises enhance urban innovation. Industrial Economics Research . https://doi.org/10.13269/j.cnki.ier.2024.02.001
Liu, M., Tan, R., & Zhang, B. (2021). The costs of “blue sky”: Environmental regulation, technology upgrading, and labor demand in China. Journal of Development Economics, 150 , 102610. https://doi.org/10.1016/j.jdeveco.2020.102610
Liu, Y. (2019). Enacting a low-carbon economy: Policies and distrust between government employees and enterprises in China. Energy Policy, 130 , 130–138. https://doi.org/10.1016/j.enpol.2019.04.001
Liu, Y. S., Cao, Y., Hou, J. J., Zhang, J. T., Yang, Y. O., & Liu, L. C. (2020). Identifying common paths of CO2 and air pollutants emissions in China. Journal of Cleaner Production, 256 , 120599. https://doi.org/10.1016/j.jclepro.2020.120599
Liu, Y., Sun, H., Meng, B., Jin, S., & Chen, B. (2023). How to purchase carbon emission right optimally for energy-consuming enterprises? Analysis based on optimal stopping model. Energy Economics, 124 , 106758. https://doi.org/10.1016/j.eneco.2023.106758
Lu, Z., Huang, L., Liu, J., Zhou, Y., Chen, M., & Hu, J. (2019). Carbon dioxide mitigation co-benefit analysis of energy-related measures in the air pollution prevention and control action plan in the Jing–Jin–Ji region of China. Resources, Conservation & Recycling: X, 1 , 100006. https://doi.org/10.1016/j.rcrx.2019.100006
Mertzanis, C., Garas, S., & Abdel-Maksoud, A. (2020). Integrity of financial information and firms’ access to energy in developing countries. Energy Economics, 92 , 105005. https://doi.org/10.1016/j.eneco.2020.105005
Mi, Z., Zhang, Y., Guan, D., Shan, Y., Liu, Z., Cong, R., Yuan, X.-C., & Wei, Y. M. (2016). Consumption-based emission accounting for Chinese cities. Applied Energy, 184 , 1073–1081. https://doi.org/10.1016/j.apenergy.2016.06.094
Mills, E. S. (1967). An aggregative model of resource allocation in a metropolitan area. The American Economic Review, 57 (2), 197–210.
Mukim, M. (2015). Coagglomeration of formal and informal industry: Evidence from India. Journal of Economic Geography, 15 (2), 329–351. https://doi.org/10.1093/jeg/lbu020
Okubo, T., Picard, P. M., & Thisse, J. F. (2010). Spatial Sorting of heterogenous firms. Journal of International Economics, 82 (2), 230–237.
Paramati, S. R., Shahzad, U., & Doğan, B. (2022). The role of environmental technology for energy demand and energy efficiency: Evidence from OECD countries. Renewable and Sustainable Energy Reviews, 153 , 111735. https://doi.org/10.1016/j.rser.2021.111735
Qi, G., Wei, W., Wang, Z., Wang, Z., & Wei, L. (2023). The spatial-temporal evolution mechanism of PM2. 5 concentration based on China’s climate zoning. Journal of Environmental Management, 325 , 116671. https://doi.org/10.1016/j.jenvman.2022.116671
Rahman, M. M., & Alam, K. (2021). Clean energy, population density, urbanization and environmental pollution nexus: Evidence from Bangladesh. Renewable Energy, 172 , 1063–1072. https://doi.org/10.1016/j.renene.2021.03.103
Ramaswami, A., Boyer, D., Nagpure, A. S., Fang, A., Bogra, S., Bakshi, B., Cohen, E., & Rao-Ghorpade, A. (2017). An urban systems framework to assess the trans-boundary food-energy-water nexus: implementation in Delhi, India. Environmental Research Letters, 12 (2), 025008. https://doi.org/10.1088/1748-9326/aa5556
Ramaswami, A., Jiang, D., Tong, K., & Zhao, J. (2018). Impact of the economic structure of cities on urban scaling factors: Implications for urban material and energy flows in China. Journal of Industrial Ecology, 22 (2), 392–405. https://doi.org/10.1111/jiec.12563
Romer, P. M. (1986). Increasing returns and long-run growth. Journal of Political Economy, 94 (5), 1002–1037. https://doi.org/10.1086/261420
Saqib, N., Ozturk, I., Usman, M., Sharif, A., & Razzaq, A. (2023). Pollution haven or halo? How European countries leverage FDI, energy, and human capital to alleviate their ecological footprint. Gondwana Research, 116 , 136–148. https://doi.org/10.1016/j.gr.2022.12.018
Shadbegian, R. J., & Gray, W. B. (2005). Pollution abatement expenditures and plant-level productivity: A production function approach. Ecological Economics, 54 (2–3), 196–208. https://doi.org/10.1016/j.ecolecon.2004.12.029
Shan, Y., Liu, J., Liu, Z., Shao, S., & Guan, D. (2019). An emissions-socioeconomic inventory of Chinese cities. Scientific Data, 6 (1), 1–10. https://doi.org/10.1038/sdata.2019.27
Shao, S., Cheng, S., & Jia, R. (2023). Can low carbon policies achieve collaborative governance of air pollution? Evidence from China’s carbon emissions trading scheme pilot policy. Environmental Impact Assessments, 103 , 107286. https://doi.org/10.1016/j.eiar.2023.107286
Sicard, P., Agathokleous, E., Anenberg, S. C., De Marco, A., Paoletti, E., & Calatayud, V. (2023). Trends in urban air pollution over the last two decades: A global perspective. Science of the Total Environment, 858 , 160064. https://doi.org/10.1016/j.scitotenv.2022.160064
Singh, J. (2005). Collaborative networks as determinants of knowledge diffusion patterns. Management Science, 51 (5), 756–770. https://doi.org/10.1287/mnsc.1040.0349
Song, M., Zhao, X., & Shang, Y. (2020). The impact of low-carbon city construction on ecological efficiency: Empirical evidence from quasi-natural experiments. The Journal Resources, Conservation & Recycling, 157 , 104777. https://doi.org/10.1016/j.resconrec.2020.104777
Sun, X., Loh, L., & Chen, Z. (2020). Effect of market fragmentation on ecological efficiency: Evience from environmental pollution in China. Environmental Science and Pollution Research, 27 , 4944–4957. https://doi.org/10.1007/s11356-019-06548-2
Tanaka, K., & Managi, S. (2021). Industrial agglomeration effect for energy efficiency in Japanese production plants. Energy Policy, 156 , 112442. https://doi.org/10.1016/j.enpol.2021.112442
Tang, P., Jiang, Q., & Mi, L. (2021). One-vote veto: The threshold effect of environmental pollution in China’s economic promotion tournament. Ecological Economics, 185 , 107069. https://doi.org/10.1016/j.ecolecon.2021.107069
Tian, Y., & Feng, C. (2022). The internal-structural effects of different types of environmental regulations on China’s green total-factor productivity. Energy Economics, 113 , 106246. https://doi.org/10.1016/j.eneco.2022.106246
Wan, Q., Chen, J., Yao, Z., & Yuan, L. (2022). Preferential tax policy and R&D personnel flow for technological innovation efficiency of China’s high-tech industry in an emerging economy. Technological Forecasting and Social Change, 174 , 121228. https://doi.org/10.1016/j.techfore.2021.121228
Wan, Q., Ye, J., Zheng, L., Tan, Z., & Tang, S. (2023). The impact of government support and market competition on China’s high-tech industry innovation efficiency as an emerging market. Technological Forecasting and Social Change, 192 , 122585. https://doi.org/10.1016/j.techfore.2023.122585
Wang, H., Li, T., Zhu, J., Jian, Y., Wang, Z., & Wang, Z. (2023a). China’s new environmental protection law: Implications for mineral resource policy, environmental precaution and green finance. Resources Policy, 85 , 104045. https://doi.org/10.1016/j.resourpol.2023.104045
Wang, K. L., Zhao, B., Ding, L. L., & Miao, Z. (2021). Government intervention, market development, and pollution emission efficiency: Evidence from China. Science of the Total Environment, 757 , 143738. https://doi.org/10.1016/j.scitotenv.2020.143738
Wang, P., & Lu, Z. (2023). Strategic interaction in environmental regulation and sulfur dioxide emissions: Evidence from China. Science of the Total Environment, 875 , 162620. https://doi.org/10.1016/j.scitotenv.2023.162620
Wang, S., & Liu, X. (2017). China’s city-level energy-related CO 2 emissions: Spatiotemporal patterns and driving forces. Applied Energy, 200 , 204–214. https://doi.org/10.1016/j.apenergy.2017.05.085
Wang, X., & Luo, Y. (2020). Has technological innovation capability addressed environmental pollution from the dual perspective of FDI quantity and quality? Evidence from China. Journal of Cleaner Production, 258 , 120941. https://doi.org/10.1016/j.jclepro.2020.120941
Wang, Y., Wei, S., He, X., & Gu, H. (2023b). Environmental regulation and entrepreneurial activity: Evidence from the low-carbon city pilot policy in China. Sustainable Cities and Society, 98 , 104829. https://doi.org/10.1016/j.scs.2023.104829
Wilkes, M. A., Carrivick, J. L., Castella, E., Ilg, C., Cauvy-Fraunié, S., Fell, S. C., Füreder, L., Huss, M., James, W., Lencioni, V., Robinson, C., & Brown, L. E. (2023). Glacier retreat reorganizes river habitats leaving Refugia for Alpine invertebrate biodiversity poorly protected. Nat Ecol Evol, 7 (6), 1–11. https://doi.org/10.1038/s41559-023-02061-5
Xu, H., Liu, W., & Zhang, D. (2023a). Exploring the role of co-agglomeration of manufacturing and producer services on carbon productivity: An empirical study of 282 cities in China. Journal of Cleaner Production, 399 , 136674. https://doi.org/10.1016/j.jclepro.2023.136674
Xu, J., Dong, Y., Xie, L., & Chen, S. (2022a). The pollution haven strikes back? Evidence from air quality daily variation in the Jing–Jin–Ji region of China. Environmental Science & Policy, 138 , 105–121. https://doi.org/10.1016/j.envsci.2022.09.014
Xu, M., Tan, R., & He, X. (2022b). How does economic agglomeration affect energy efficiency in China? Evidence from endogenous stochastic frontier approach. Energy Economics, 108 , 105901. https://doi.org/10.1016/j.eneco.2022.105901
Xu, S., Wang, X., Zhu, R., & Wang, D. (2023b). Spatio-temporal effects of regional resilience construction on carbon emissions: Evidence from 30 Chinese provinces. Science of the Total Environment, 887 , 164109. https://doi.org/10.1016/j.scitotenv.2023.164109
Yan, Y., & Huang, J. (2022). The role of population agglomeration played in China’s carbon intensity: A city-level analysis. Energy Economics, 114 , 106276. https://doi.org/10.1016/j.eneco.2022.106276
Ye, C., Sun, C., & Chen, L. (2018). New evidence for the impact of financial agglomeration on urbanization from a spatial econometrics analysis. Journal of Cleaner Production, 200 , 65–73. https://doi.org/10.1016/j.jclepro.2018.07.253
Yu, Q., Li, M., Li, Q., Wang, Y., & Chen, W. (2022a). Economic agglomeration and emissions reduction: Does high agglomeration in China’s urban clusters lead to higher carbon intensity? Urban Clim, 43 , 101174. https://doi.org/10.1016/j.uclim.2022.101174
Yu, Y., Dai, C., Wei, Y., Ren, H., & Zhou, J. (2022b). Air pollution prevention and control action plan substantially reduced PM2. 5 concentration in China. Energy Economics, 113 , 106206.
Zeng, D. Z., & Zhao, L. (2009). Pollution havens and industrial agglomeration. Journal of Environmental Economics and Management, 58 (2), 141–153. https://doi.org/10.1016/j.jeem.2008.09.003
Zhang, B., Yu, L., & Sun, C. (2022a). How does urban environmental legislation guide the green transition of enterprises? Based on the perspective of enterprises’ green total factor productivity. Energy Economics, 110 , 106032. https://doi.org/10.1016/j.eneco.2022.106032
Zhang, L., He, X., & Jia, Z. (2023). Industrial agglomeration, public services and city size: Evidence from 286 cities in China. Land Use Policy, 131 , 106758. https://doi.org/10.1016/j.landusepol.2023.106758
Zhang, L., Sun, H., Pu, T., Sun, H., & Chen, Z. (2024). Do green finance and hi-tech innovation facilitate sustainable development? Evidence from the Yangtze River Economic Belt. Economic Analysis and Policy, 81 , 1430–1442. https://doi.org/10.1016/j.eap.2024.02.005
Zhang, M., & Liu, Y. (2022). Influence of digital finance and green technology innovation on China’s carbon emission efficiency: Empirical analysis based on spatial metrology. Science of the Total Environment, 838 , 156463. https://doi.org/10.1016/j.scitotenv.2022.156463
Zhang, M., Wang, L., Ma, P., & Wang, W. (2022b). Urban-rural income gap and air pollution: A stumbling block or stepping stone. Environmental Impact Assessments, 94 , 106758. https://doi.org/10.1016/j.eiar.2022.106758
Zhao, C., & Wang, B. (2022). How does new-type urbanization affect air pollution? Empirical evidence based on spatial spillover effect and spatial Durbin model. Environment International, 165 , 107304. https://doi.org/10.1016/j.envint.2022.107304
Zhao, H., & Lin, B. (2019). Will agglomeration improve the energy efficiency in China’s textile industry: Evidence and policy implications. Applied Energy, 237 , 326–337. https://doi.org/10.1016/j.apenergy.2018.12.068
Zhou, D., Qiu, Y., & Wang, M. (2021). Does environmental regulation promote enterprise profitability? Evidence from the implementation of China’s newly revised Environmental Protection Law. Economic Modelling, 102 , 105585. https://doi.org/10.1016/j.econmod.2021.105585
Zhu, Q., Li, X., Li, F., Wu, J., & Zhou, D. (2020). Energy and environmental efficiency of China’s transportation sectors under the constraints of energy consumption and environmental pollutions. Energy Economics, 89 , 104817. https://doi.org/10.1016/j.eneco.2020.104817
Download references
Acknowledgements
This work was supported by the National Social Science Foundation of China (Grant No. 21XRK007); Excellent Doctoral Student Research Innovation Project for Xinjiang University (Grant No. XJU2022BS008). The graduate research and innovation project of Xinjiang Autonomous Regions (Grant No. XJ2024G010).
Author information
Authors and affiliations.
School of Economics and Management, Xinjiang University, Urumqi, 830002, Xinjiang, People’s Republic of China
Jiamin Liu, Xiaoyu Ma, Jiaoning Zhang & Qiuqiu Guo
Xinjiang Innovation Management Research Center, Xinjiang University, Urumqi, 830002, Xinjiang, People’s Republic of China
School of Economics and Management, Guangxi Normal University, Guilin, 541006, People’s Republic of China
You can also search for this author in PubMed Google Scholar
Contributions
JL: conceptualization, writing-original draft, formal analysis. XM: methodology, writing-review, editing, formal analysis. JZ: Data curation, Writing-review and editing, Methodology. CH: methodology, visualization, software. QG: data curation, methodology.
Corresponding author
Correspondence to Xiaoyu Ma .
Ethics declarations
Conflict of interest.
We declare that we do not have any commercial or associative interest represents a conflict of interest in connection with the work submitted.
Additional information
Publisher's note.
Springer Nature remains neutral with regard to jurisdictional claims in published maps and institutional affiliations.
Rights and permissions
Springer Nature or its licensor (e.g. a society or other partner) holds exclusive rights to this article under a publishing agreement with the author(s) or other rightsholder(s); author self-archiving of the accepted manuscript version of this article is solely governed by the terms of such publishing agreement and applicable law.
Reprints and permissions
About this article
Liu, J., Ma, X., Zhang, J. et al. Air pollution mitigation and CO 2 emission reduction effects of heterogeneous enterprises synergistic agglomeration. Environ Dev Sustain (2024). https://doi.org/10.1007/s10668-024-05497-2
Download citation
Received : 22 April 2024
Accepted : 01 October 2024
Published : 07 October 2024
DOI : https://doi.org/10.1007/s10668-024-05497-2
Share this article
Anyone you share the following link with will be able to read this content:
Sorry, a shareable link is not currently available for this article.
Provided by the Springer Nature SharedIt content-sharing initiative
- Manufacturing enterprises
- Productive service enterprises
- Heterogeneity
- Synergistic agglomeration
- Air pollution
- CO 2 emissions
- Find a journal
- Publish with us
- Track your research
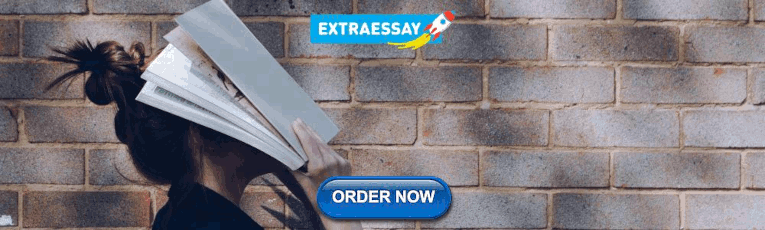
IMAGES
VIDEO
COMMENTS
Formulating a Hypothesis Write a hypothesis about the effect of the fan speed on the acceleration of the cart. Use the "if . . . then . . . because- . ." format and be sure to answer the lesson question: "How does an object's position and velocity change as the object accelerates?"
Final answer: The effect of the fan speed on the cart's acceleration could be that as the fan speed increases, the cart's acceleration also increases due to a more significant force exerted by the fan.When an object accelerates, its position and velocity change more rapidly. Explanation: A possible hypothesis regarding the fan speed and the acceleration of the cart might be: 'If the fan speed ...
The fan laws were derived from dimensionless analysis of the equations for volumetric flow rate, static pressure head, and power as a function of fan diameter, air density and rotational speed. The purpose of this study is to characterize a fan's performance characteristics at various rotational speeds and ambient pressures.
There is no formula to calculate the required fan speed, but if a specific fan configuration is known then fan similarity laws can be used to calculate performance based on known performance of a similar fan. For example, for a given fan design the flow varies linearly with speed, static pressure varies with the square of the speed, and power ...
Write a hypothesis about the effect of the fan speed on the acceleration of the cart. Use the "if . . . then . . . Sample Response: If the fan speed increases, then because . . .'' format and be sure to answer the lesson the acceleration increases because a greater fan speed question: "How does an object's position and velocity supplies more energy to move the cart. change as the object ...
See Speed control for PSC induction motor As illustrated in that question, it is a natural characteristics of a fan that it requires less torque to operate at lower speeds. That mean that less power is used, since power is torque multiplied by speed. An increased proportion of that power is dissipated in the rotor of the motor, but less total ...
System Effect and Its Impacts. System effect refers to losses in air-system performance caused by adverse flow conditions (excess turbulence or swirl) at or near the fan. System effect can occur at a fan's inlet or outlet or both. Often, it results from changes to system design—commonly involving the length, width, and/or transition points ...
Write a hypothesis about the effect of the fan speed on the acceleration of the cart. Use the "if . . . then . . . because . . .†format and be sure to answer the lesson question: "How does an object's position and velocity change as the object accelerates? Write a hypothesis about the effect of the fan speed on the acceleration of the cart.
In this study, the effects of the jet fan speed, heat release rate and aspect ratio on smoke movement in tunnel fires have been investigated. The jet fan speed was changed from 6.25 (25%) to 12.5 m/s (50%), 18.75 m/s (75%), and 25 m/s (100%). The heat release rate was set up from 3.9 to 6 MW and 16 MW, the aspect ratio was changed from 0.6 to 1 and 1.5, respectively. The lower the jet fan ...
To start we will consider only the effect of a change in the speed of the fan on: Volume flow rate/volume of air; Pressure; Power consumption . Assumptions Used in These Examples: We will assume that the fan size and air density are to remain constant. The first three derivations of the Fan Laws are predicated on a couple of assumptions:
1) Airflow is directly proportional to fan speed. If fan speed is reduced by 10%, the airflow rate will decrease by 10%. 2) Pressure is proportional to the fan speed squared, if the fan speed is reduced by 10%, pressure will decrease by 19%. 3) Fan energy consumption is proportional to the fan speed cubed. If the fan speed is reduced by 10%,
Natural ventilation associated with a mechanical fan is a feasible strategy to enhance thermal acceptability in warm weather. The ASHRAE-55 provides the increase for operative temperature proportional to the increase in air speed while maintaining thermal comfort. Conversely, the range of informed values is limited and little guidance for mechanical fans is provided. This work explores the ...
Yes, you better believe that fan speed will affect the AC. The fan is an integral component of any HVAC system. How it operates will greatly affect the outcome of the AC. To start, it is important to realize that a residential or commercial air conditioning system contains two fans. They would be the outdoor fan (condenser fan) or the indoor ...
fans to save energy are of great importance for fan man-ufacturers. Fans transfer static and kinetic energy to the air, creating a pressure difference that causes the air to flow. Fan efficiency is often misunderstood due to the variety of definitions of a fan and what contributes to the losses in a N. Dizadji (&) A. M. Mahmoudkhani N. Nouri
If the fan speed increases, then the acceleration of the cart will also increase because air resistance will be higher, resulting in a greater force pushing against the cart. The effect of an object's position and velocity on its acceleration is that as an object accelerates, its velocity increases, and its position changes.
Beneficial effect of electric fans in extreme heat and humidity. ScienceDaily . Retrieved October 6, 2024 from www.sciencedaily.com / releases / 2015 / 02 / 150217114003.htm
Formulating a Hypothesis Write a hypothesis about the effect of the fan speed on the acceleration of the cart. Use the "if then because "format and be sure to answer the lesson question: "How does an object's position and velocity change as the object accelerates?"
hypothesis Write a hypothesis about the effect of the fan speed on the acceleration of the cart. Use the 'if . . . then . . . because . . .' format and be sure to answer the lesson question: "How does an object's position and velocity change as the object accelerates?"
If the fan speed increases, then the acceleration of the cart also increases, because the force exerted by the fan on the cart becomes greater. As a result, the cart's velocity increases more rapidly over time, leading to a greater change in position.
Click here to get an answer to your question: Write a hypothesis about the effect of the fan speed on the acceleration of the cart. Use the "if . . then ... because ...
Formulating a Hypothesis Write a hypothesis about the effect of the fan spood on the acceleration of the cart Use the "if __ then __ because __ format and be sure to answer the lesson question: "How does an object's position and velocity change as the object accelorates? square
Write a hypothesis about the effect of the fan speed on the acceleration of the cart. Use the "if . . . then . . . because . . ." format and be sure to answer the lesson question: "How does an object's position and velocity change as the object accelerates?"
If the fan speed increases, then the acceleration increases because a greater fan speed supplies more energy to move the cart. Simplify. Explain. ... Write a hypothesis about the effect of increasing the total mass of the carts on the final velocity after an inelastic collision. Use the "if . . . then . . . because . . ."
Therefore, the effects of Msa on Aip and CO 2 may be hedged due to the "market congestion" effect. Hypothesis H4 is proposed: Hypothesis H4. As the market potential gradually expands, the impact of Msa on Aip and CO 2 shows obvious characteristics: promotion→inhibition→ineffectiveness. 3.4 Shock effects of environmental protection policy