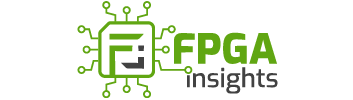
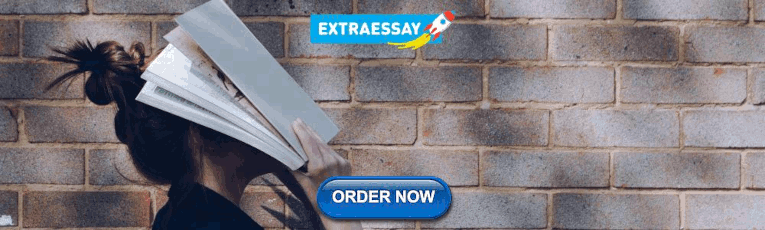
System Verilog Operators: A Comprehensive Guide
Niranjana R
December 3, 2023
System Verilog operators : is a hardware description language used in the development and verification of digital circuits and systems. Similar to other programming languages, it has a set of operators that allow designers to perform various operations on the data. System Verilog operators are classified into different categories based on their functionality. Understanding these operators is crucial for designing efficient and error-free digital circuits.
The basic System Verilog operators include arithmetic, relational, equality, logical, bitwise, and shift operators. These operators are used to perform basic mathematical and logical operations, such as addition, subtraction, multiplication, division, and comparison, and logical operations like AND, OR, and NOT. Advanced System Verilog operators include reduction, concatenation, replication, and streaming operators. These operators are used to perform complex operations on large data sets, such as data compression, encryption, and decompression.
Table of Contents
Key Takeaways
- System Verilog operators are used in the development and verification of digital circuits and systems.
- Basic System Verilog operators include arithmetic, relational, equality, logical, bitwise, and shift operators.
- Advanced System Verilog operators include reduction, concatenation, replication, and streaming operators.
Basic System Verilog Operators
In System Verilog, operators are essential building blocks for designing digital circuits. These operators allow us to perform various operations on digital data and manipulate it to achieve the desired output. In this section, we will discuss the three basic types of operators: Arithmetic, Relational, and Logical.
Arithmetic Operators
Arithmetic operators are used to perform mathematical operations on operands. The basic arithmetic operators in System Verilog are:
For example, the expression a = b + c adds the values of b and c and stores the result in a.
Relational Operators
Relational operators are used to compare two operands. The result of a relational operator is either true or false. The relational operators in System Verilog are:
For example, the expression if (a == b) compares the values of a and b and executes the code inside the if statement if they are equal.
Logical Operators
Logical operators are used to perform logical operations on operands. The logical operators in System Verilog are:
For example, the expression if (a && b) checks if both a and b are true and executes the code inside the if statement if they are.
Understanding these basic operators is essential for designing digital circuits in System Verilog. By using these operators, we can perform various operations on digital data and manipulate it to achieve the desired output.
Advanced System Verilog Operators
In addition to the basic operators, System Verilog provides advanced operators to perform complex operations. These operators are an essential part of System Verilog and are widely used in the development of digital circuits. In this section, we will discuss two of the most important advanced operators: Bitwise Operators and Shift Operators.
Bitwise Operators
Bitwise operators are used to manipulate individual bits of a variable. System Verilog provides six bitwise operators: AND, OR, XOR, NAND, NOR, and XNOR. These operators can be used to perform various operations such as masking, setting, and clearing bits.
Shift Operators
Shift operators are used to shift the bits of a variable left or right. System Verilog provides four shift operators: left shift, right shift, arithmetic right shift, and logical right shift. These operators are used to perform various operations such as multiplication and division by powers of two.
In summary, bitwise and shift operators are essential in System Verilog for manipulating individual bits and shifting bits left or right. By using these advanced operators, we can perform complex operations with ease and efficiency.
Assignment Operators in System Verilog
In System Verilog, we use assignment operators to assign values to variables. The most commonly used assignment operator is the “=” operator. We use this operator to assign a value to a variable. For example, if we want to assign the value of 5 to a variable named “a” , we would write “a = 5;” .
System Verilog also provides us with other assignment operators that we can use to perform certain operations on variables while assigning values to them. These operators are listed in the table below:
By using these assignment operators, we can perform operations on variables and assign the result to the same variable in a single statement. This makes our code more concise and easier to read.
Conditional Operators in System Verilog
In System Verilog, conditional operators are used to create conditional expressions. They are a shorthand way to write if/else statements. The conditional operator is also known as the ternary operator because it takes three operands. The syntax of the conditional operator is as follows:
The conditional operator evaluates the condition first. If the condition is true, it evaluates the expression immediately after the question mark. If the condition is false, it evaluates the expression immediately after the colon.
One of the advantages of using the conditional operator is that it makes the code more concise and easier to read. It can also be used in assignments, which can help reduce the number of lines of code.
Another conditional operator in System Verilog is the implication operator. The implication operator is used to create logical implications. It takes two operands and returns a Boolean value. The syntax of the implication operator is as follows:
The implication operator returns true if the condition is false or if both the condition and the expression are true. It returns false if the condition is true and the expression is false.
In summary, System Verilog provides two types of conditional operators: the conditional operator and the implication operator. These operators can help reduce the number of lines of code and make the code more concise and easier to read.
Miscellaneous Operators in System Verilog
In addition to the basic arithmetic, relational, and logical operators, System Verilog provides several miscellaneous operators that can be used in digital circuit design.
Replication Operator
The replication operator {} is used to replicate a single value or a set of values multiple times. The syntax for the replication operator is {N{value}}, where N is the number of times the value is replicated.
For example, {8{1’b0}} represents a vector of eight bits, all set to 0. This operator is useful when designing circuits that require a large number of identical components.
Concatenation Operator
The concatenation operator {} is used to concatenate two or more vectors into a single vector. The syntax for the concatenation operator is {vector1, vector2, …, vectorN}, where vector1 through vectorN are the vectors being concatenated.
For example, {a, b} represents a vector that is the concatenation of vectors a and b. This operator is useful when designing circuits that require combining multiple vectors into a single vector.
Ternary Operator
The ternary operator ?: is a conditional operator that is used to assign a value to a variable based on a condition. The syntax for the ternary operator is condition ? true_value : false_value, where condition is the condition being evaluated, true_value is the value assigned if the condition is true, and false_value is the value assigned if the condition is false.
For example, y = (x>0) ? 1 : 0 assigns the value 1 to y if x is greater than 0, and assigns the value 0 to y if x is less than or equal to 0. This operator is useful when designing circuits that require conditional assignments.
Bit-select and Part-select Operators
The bit-select operator [] and the part-select operator [start:end] are used to select a single bit or a range of bits from a vector, respectively. The syntax for the bit-select operator is vector[index], where vector is the vector being selected from and index is the index of the bit being selected. The syntax for the part-select operator is vector[start:end], where vector is the vector being selected from, start is the index of the starting bit, and end is the index of the ending bit.
For example, a[7:0] represents a vector that consists of the eight least significant bits of vector a. This operator is useful when designing circuits that require selecting specific bits or ranges of bits from a vector.
These operators, along with the basic operators, provide a powerful set of tools for designing digital circuits using System Verilog.
Related Articles
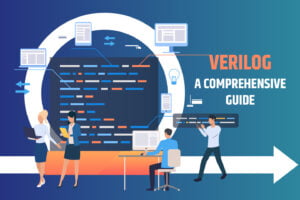
Leave a Comment
This site uses Akismet to reduce spam. Learn how your comment data is processed .
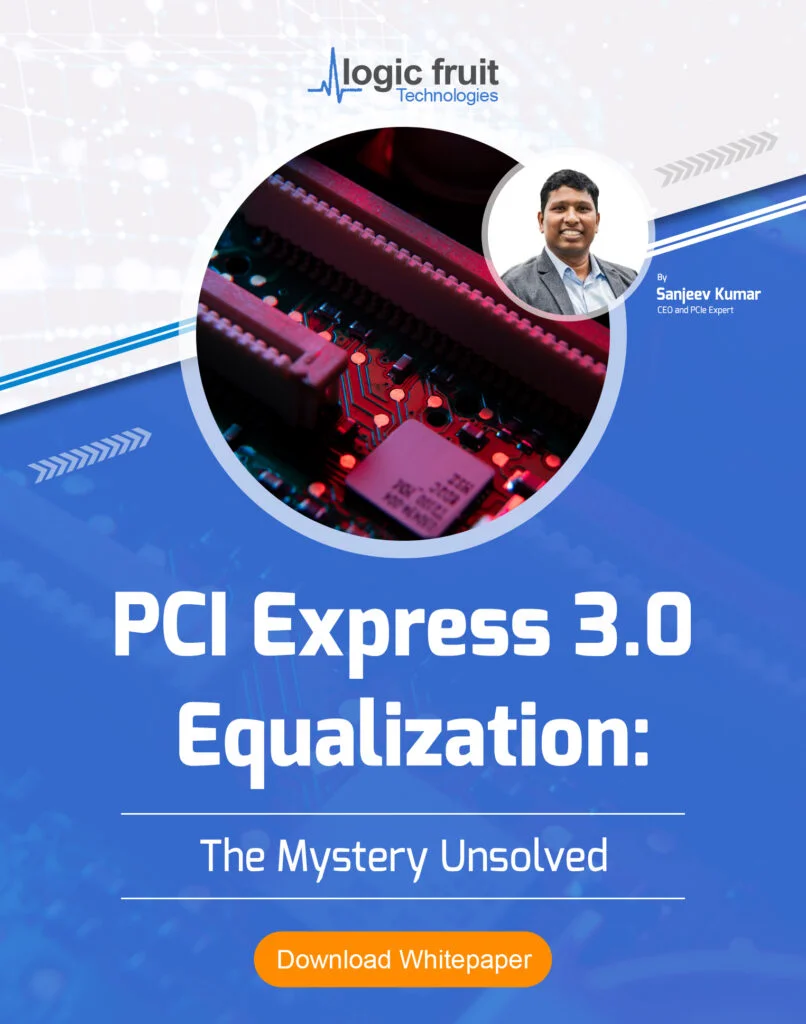
most recent
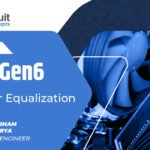
Logic Fruit Technologies Releases Next-Generation PCIe Gen6 Retimer Equalization Technology
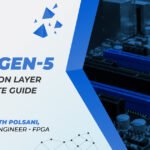
Logic Fruit’s Latest Whitepaper Unveils PCIe Gen-5 Secrets for High-Speed Data Transfer
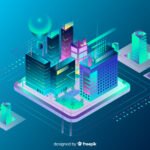
Artificial Intelligence
Ai in smart cities: advancing urban sustainability.
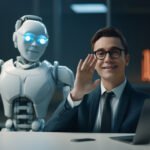
AI-Powered Customer Service: Virtual Assistants & Chatbots
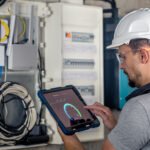
Power Management
Empowering real-time power management with smart metering.
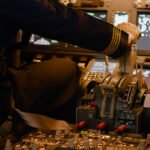
Test & Measurement
Precision & reliability in aerospace: the test & measurement role, subscribe to get our best viral stories straight into your inbox, related posts.
- Loops in Verilog: A Comprehensive Guide November 23, 2023
- FPGA-Based Robotics and Automation August 28, 2023
- Exploring System Verilog's If-Else Constructs: A Comprehensive Guide December 27, 2023
- PCIe 6.0 - Beginner's Guide to PCIe 6.0 September 27, 2023
- Mastering SystemVerilog Arrays: A Comprehensive Guide December 27, 2023
- FPGA in Space Exploration: Powering Satellites and Spacecraft November 24, 2023
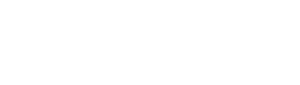
FPGA Insights have a clear mission of supporting students and professionals by creating a valuable repository of FPGA-related knowledge to contribute to the growth and development of the FPGA community and empower individuals to succeed in their projects involving FPGAs FPGA Insights has been producing FPGA/Verilog/VHDL Guides and blogs with the aim of assisting students and professionals across the globe. The mission of FPGA Insights is to continually create a growing number of FPGA blogs and tutorials to aid professionals in their projects.
© 2024 Fpga Insights. All rights reserved
Beyond Circuit Podcast by Logic Fruit: High-speed video interfaces in Indian Aerospace & Defence.
Modules, Controls, and Interfaces
3.1 modules.
Modules are the basic building blocks of SystemVerilog. It is intended to be a reusable component that can be connected to form a larger component. To declare a module, we can use the following syntax:
Notice that due to legacy reason, there is no namespace for modules. As a result, module_name has to be unique in your entire design. To declare the ports for the module, we can simply do
Keywords input and output are used to specify the direction of ports. There is another keyword, inout which makes the port bidirectional. inout is typically used for tri-state designs and we will not cover it in the book. If you are declaring multiple ports sharing the same port direction and types, you can omit the subsequential ones, as shown below. Notice that the code is equivalent. It is up to the designers to choose which style they want to follow. In this book we will use the more verbose version.
The type for the ports can be any integral values, such as logic, arrays, or struct. It can also be interface, which will be covered later in the chapter.
Notice that there is another style of declaring port, which is specifying port names first, then later on declare the port direction and types, typically called Non-ANSI style. This style is out-dated and we do not recommend use it in practice.
To declare variables inside the module, we can simply put definition inside the module endmodule .
In the example code we declare 8-bit value value . Notice that it is highly recommended to declare the variable type before using the variable. Although implicit logic declaration is supported in SystemVerilog, it is dangerous and usually triggers compiler warnings/errors.
3.1.1 Module Parameters
SystemVerilog allows the module definition parametrized by certain values, which makes the modules more reusable. For instance, suppose we have an ALU module parametrized by the data width, we can reuse the same definition for both 32-bit and 64-bit ALU instantiation.
To declare a parametrized module, we can use the following syntax, which is also the ANSI style.
In the example above, module mod_param is parametrized by two parameters WIDTH and VALUE . We immediately use WIDTH to parametrize the bit-width of in and out . Notice that we also specify the data type for VALUE . In general we recommend to specify the data type of a parameter. If it used for data width parametrization, int should suffice. In the example we also give the parameters a default value, which is highly recommended to do so.
There is another type of “parameter” called localparam . It is not parameterization per se, since its value cannot be changed through instantiation. However, for the sake of completeness we will cover it here. Local parameters are typically used for storing magic numbers. For state values, however, you should use enum instead.
In the example above we define a magic number VALUE to have the value of 42. We can later use VALUE whenever we need its value.
3.1.2 Module Instantiation and Hierarchy
Once we have a module definition, we can instantiate it in a parent module. Suppose we have a module definition as follows:
We can instantiate the child module as follows:
In the example above, we first declares three variables, clk , in , out , which will be wired to our child instance. To instantiate the child module, we create an instance called child_inst . To specify the port wiring, we use .child_port_name(parent_var_name) syntax. It means to wire parent_var_name from the parent module to child_port_name port from the child instance.
There is another short-hand to instantiate the child module in our case. Since the child_port_name is identical to parent_var_name , we can do the following
(.*) tells the compiler to automatically find and wire matching variable from the parent module. You can even override the default matching with extra connections, as shown below, which wires clk_in to child_inst ’s clk and leaves the rest to the default matching.
Although it may simplify the code and make it more readable, because the matching only relies on the name, it may be matched to an unexpected wire. We recommend to only use this style when the design is simple.
To instantiate a module with different parameter values other than the default ones, we can do the following, using the module mod_param defined earlier.
In the example above we override the parameter value WIDTH with 16. Notice that we have to manually change the bit-width of in and out . A better way to do is the following, where the bit-width is only specified by a single parameter in the parent scope.:
To access variables through hierarchy, we can do child_inst.out from the parent module. We only recommend to do so in test bench, in instead of RTL code for synthesis.
A design style where all the logic are specified through module instantiation rather than the procedural blocks is called structural Verilog. Unless you are very experienced in RTL design or have a particular need in physical design, we highly recommend not to use such style in RTL design. It will reduce the synthesis quality and make verification more difficult. We will discuss the benefit of another style, behavioral Verilog, where design logics are specified through procedural blocks.
3.2 Continuous Assignment
Continuous assignment wires the values on the right hand side to the left side. Continuous in its name implies that whenever an operand in the right-hand expression changes, the whole right-hand side expression shall be evaluated and its result will be assigned to the left hand side. This is used to model combinational circuit where the output of the circuit updates its value whenever the input values change.
To use continuous assignment, we can do
You can of course use more complex expression such as
There are couple rules apply to continuous assignments:
- Continuous assignment can only appear in the scope of a module, that is, its lexical parent should be module . You cannot declare a continuous assignment in other scopes such as procedural blocks or functions, which we will cover shortly.
Each bit of left hand side can only be assigned to once as continuous assignments. For instance, it is illegal to do something below, where bit a[1] is assigned twice.
The left hand can only be a net/variable, or a select of a vector/net, or a concatenation. For the case of concatenation, the operator can be seen as “unpacked” in the concatenation order, as shown below, where the sum of a , b , and cin is split into cout and sum . Since cout is only 1-bit, it gets the value of carry out. ```SystemVerilog // a 4-bit adder with carry logic [3:0] a; logic [3:0] b; logic [3:0] sum; logic cin; logic cout;
assign {cout, sum} = a + b + cin; Notice you can also perform a continuous assignment when declaring a variable as initialization, as below: SystemVerilog logic [3:0] d = 4’h1; Although it works well for ASIC with constant initialization, it will only work with a subset of FPGA boards and you shall check the targeted compiler when using this syntax. We recommend not to use this syntax if the code is intended to be portable. However, the following syntax: SystemVerilog logic a = b & c; ``` may not be synthesizable for some synthesis tools. We highly recommend to use continuous assignment for this use case.
3.3 Procedural Blocks
Procedural blocks, also known as processes , are the building blocks for a module’s logic. There are five different procedural blocks:
- initial procedure
- always_comb
- always_latch
- final procedure
We will cover each procedural blocks in details in a slightly different order. We will not cover the Verilog-95 procedural block always here since it is out-dated and error-prone compared to the new syntax.
3.3.1 always_comb : Modeling Combination Logic
The keyword always_comb denotes the combinational nature of the procedure block: every logic contained inside the block will be synthesized into combinational circuits. The general syntax for always_comb is shown below:
begin and end are needed if there are more than one statements in the block. If there is only one statement, we can drop the begin and end , e.g.
It is up to the design style in your project whether such syntax is allowed. In this bool we will use begin and end regardless of the number of statements inside the block.
There are several rules applies to always_comb :
- Similar to assign , the bits on the left hand side can only assigned in a single always_comb . Some simulator may not error out when there is multiple always_comb blocking assigning to the same bit, but that is undefined behavior. You cannot mix the bit assignment with other procedural blocks either.
- The evaluation of each statement is in-order. The simulator will go through each statement from top to bottom and evaluate them.
The simulator will re-evaluate the block whenever a variable on the right-hand side changes. However, there are several exceptions. One major exception is that there is no “self-triggering”. When the variable both exists on the left hand and right hand side, updating that variable will not trigger re-evaluation, as shown below:
- One benefit of using always_comb is that it forces synthesis tool to check your code based on the design intention. If any variable inside always_comb is inferred as a latch, the tool shall issue a warning or error. We will discuss under which condition latch inference happens when we introduce conditional control constructs.
- always_comb is also sensitive to the contents of a function, which we will cover shortly.
In simulator, the simulator will evaluate the always_comb once after the initial and always procedures have been started.
3.3.2 always_latch : Modeling Latched Combinational Logic
The always_latch construct functionally is identical to always_comb except for the fact that it tells the synthesis tools to check whether enclosed logic presents latched logic. All the other rules applied to always_comb are applicable to always_latch .
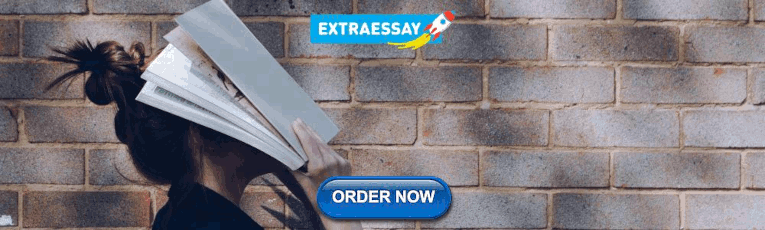
3.3.3 always_ff : Modeling Sequential Logic
The syntax for always_ff is shown below:
The signal list inside @() is called sensitivity list, which tells the synthesis tools that the signal updates are triggered by the sensitivity list. Keyword posedge implies that the procedure shall be evaluated at the positive (rising) edge of the signal, and negedge implies the negative (falling) edge of the signal. All the signals in the sensitivity list should be 1-bit.
For RTL design, there are generally two different ways to implement a reset, i.e. synchronous reset and asynchronous reset. The are mainly distinguished by whether to include reset signal in the always_ff sensitivity list. If reset signal is included, then it is asynchronous reset, meaning the reset can happen independently of the clock. In ASIC design, there are advantage and disadvantages of using asynchronous reset:
- Asynchronous reset can result in cleaner data path, if the technology library has async-reset flip-flops, which is typically the case. This implies that we can push the limit for data-path timing.
- Because of the additional triggering of reset signal, asynchronous reset results in slightly bigger circuits. However, in a modern ASIC design where there are billion gates, adding one or two gates to each register is not a big problem.
- For asynchronous design, if the assertion/de-assertion of reset signal is close to the clock edge, the circuit will go to a metastable state, and as a result the reset state could be lost.
Whether to use synchronous or asynchronous reset depends on your design needs and style guide, as long it is used consistently. In this book we will use asynchronous reset whenever necessary.
Another aspect of the reset is posedge/negedge reset. If negedge is used in the sensitivity list, it is said to reset low , and reset high for posedge . Due to some legacy reasons, modern ASIC technology only offer registers with reset low. As a result, if the design uses posedge reset, an inverter gate will be used with the standard cell. Again, adding one gate for each register is not that much an issue when modern ASIC designs. Whether to use reset high or low depends on your style guide. In this book we will use reset low.
Notice that due to naming convention, if the reset is reset low, we usually suffix _n at the end of the signal name to signify that it is negedge reset, e.g., rst_n , reset_n . In this book we will follow this convention.
In additional to the sensitivity list, always_ff also uses a special assignment called nonblocking assignment . Contract to normal assignment, called blocking assignment where = is used, nonblocking assignment uses <= . All the assignments in always_ff should be nonblocking assignment, and nonblocking assignment can only be used inside always_ff , for synthesis purpose. Although mixing blocking and nonblocking assignments is allowed in test bench code, it is strongly discouraged.
The simulation semantics for nonblocking assignment is also different from blocking assignment. As the name suggests, the value update is not “blocking”, that is, the left hand side is not updated immediately, as shown in the example below.
In the always_ff block, when the simulator evaluate the first assignment a <= b , it will evaluate the right hand side first, store the result value internally, and then proceed to the next statement. After every statement is evaluated, the simulator will update the left hand side at the same time . Hence a will get b ’s value before the clock edge and b gets a ’s.
In the always_comb block, however, the simulator will update the left hand side immediately after evaluating the right hand side, before going to the next statement, hence blocking . In this case, after the first assignment, both a and b will be 1.
This nonblocking assignment simulation semantic is designed to mimic the actual physical behavior. In the physical circuit, as long as there is no timing violation, at the clock edge, the flip-flop will take whatever values on its input wires and do an update. It does not care about whats the immediate value between the clock edges. If you wire two flip-flops in a loop, as shown in the example, at the clock edge, the flip-flop can only grab each other’s old value, since the update has not happened yet.
This semantics also allows priority coding in always_comb , as shown below:
Since it is blocking assignment, although after the first statement, a becomes 0, after the second assignment, a is re-assigned to b . This kind of coding style is perfectly legal and sometimes preferred, as we will discuss in the book.
However, if you do that in always_ff with non-blocking assignment, the result is undetermined. Different simulators and synthesis tools may have different interpretation and you may see inconsistent simulation and synthesis result. This kind of usage should be prohibited.
Similar to other always blocks, variable can only be assigned inside the same always_ff block.
3.3.4 initial Procedure
An initial procedure will execute when the simulator starts and will only execute once. In ASIC design, initial procedure is not synthesizable and will be ignored during synthesis - most synthesis tools will report a warning.
The most common way to use initial procedure is for test bench, where stimulus are provided in initial procedure to drive the simulation. We will discuss more in details when we discuss test bench design.
An example of initial is provided below:
3.3.5 final Procedure
Similar to initial procedure, final will be executed at the end of simulation and will only be executed once. If there are multiple final procedures, they will be executed in arbitrary order. final procedures are usually used for display simulation statistics or cleaning up the simulation environment.
3.3.6 Functions
Similar to C/C++, functions in SystemVerilog allows designers to reuse useful logic. The syntax for function is shown below:
For functions that has return type, keyword return must to be used to indicate return value. In old Verilog-95, return value can be assigned via function_name = return_value; . This style is outdated and we will use keyword return instead.
There is another style of writing functions that allows multiple outputs:
In the example above, logic b and c will be assigned after the function call. This is similar to reference arguments in C++.
If your function is recursive, keyword automatic is needed so that the tools will allocate separate stack space when simulate. We will discuss the reasoning when we introduce the variable scoping rules.
Functions in SystemVerilog is synthesizable with certain restrictions:
- Functions cannot have any timing controls statements, such as details and semaphore, or any calls to constructs that have timing controls statements, such as tasks.
- Recursive functions must be able to fully elaborate during synthesis. Synthesis tools typically inline function and unroll the recursion. Undetermined recursion does not guarantee a finite and fixed number of recursion, thus cannot be realized into hardware. This is similar to recursive template in C++, where the template expansion happens during compilation.
To call the function, there are general two ways:
Style 1 is similar to function calls in other software programming languages and style 2 is similar to module instantiation in SystemVerilog. In general, if the function only has a few arguments and does not use input / output in their function signature, we will use style 1, and style 2 otherwise.
If the return value of a function call is not needed, most compilers will issue a warning or error. We need to cast the return value to void to avoid this issue:
3.3.7 Tasks
Tasks are very similar to function except the following things:
- Tasks allow timing controls in their enclosed logic. The timing control can be delay, fork, and other statements.
- Tasks do not have a return type.
Although some synthesis tools might be able to synthesize tasks that do not have timing control statements, we highly recommend you to use functions for RTL design, and tasks for simulation and verification.
The general syntax for task is shown below:
3.4 Procedural Statements
Procedural statements, as the name suggests, can only exist inside the procedural blocks such as always_comb and function . There are many types of procedural statements and we will cover the following types:
- Selection statement: if and case statement
- Loop statements: for and while
- Jump statements: continue , and break
We will cover loop and jump statement together since they are often used together.
3.4.1 if Statement
The syntax for if statement is shown below
Whether to omit begin ... end when there is only one statement depends on the style guide. In this book we will omit begin ... end whenever it makes code easier to read.
Although expr can actually be a multi-bit expression, since the condition is evaluated against zero, it is generally suggested to make it 1-bit. For instance,
should be written as
for clarity. Like C/C++, dangling else can also be a problem when begin ... end is omitted for nested if statement. In this case we suggest you always use begin ... end block.
3.4.1.1 Additional Keywords for if Statement
SystemVerilog offers several keywords to if statement can be useful to check the correctness of implementation:
unique . An error will be issued during simulation if no condition matches unless there is an else statement. For instance
However, if unique0 is used, there will be any violation report. In general, use unique0 whenever there are some cases not covered by the conditions, but serve no logic.
priority explicitly tells tools that if there is overlaps in the conditions, use the lexically precedent condition first. This is useful to prevent inconsistent behavior between simulators and synthesis tool, where the simulator by default checks conditions in order whereas the synthesis may compile a parallel circuit due to synthesis macros or commands. This ensures the designer’s intent get passes to various tools in a consistent manner.
These keywords are introduced to remove inconsistency between simulator and synthesis tools. Unless explicitly specified in the design style guide, we recommend use these keywords as much as possible. However, in some cases, this keywords may increase the workload for formal verification tools, thus prohibited in some design companies. Again, these keywords usage depends on your project specific design style guide.
3.4.1.2 Latch Created from if Statement
A latch is created if the logic’s value depends on its previous value. If not specified properly, variables used inside if statement will be inferred as a latch during synthesis, resulting in undesired behavior. In the example below, we create a latch unintentionally:
If a = 1 , we will have b = 1 . However, if a = 0 , b will not be set, thus retaining its old value. As a result, we have created latch a . Notice that since we are using always_comb keyword, synthesis will report either a warning or error once a latch is inferred. There are usually two ways to solve latch issue:
- Specify a default value at the beginning of the always_comb block: ```SystemVerilog always_comb begin b = 0; if (a) b = 1; end
- Fully specify the if conditions: SystemVerilog always_comb begin if (a) b = 1; else b = 0; end
Choosing which one to use depends on the logic. Sometimes setting default value makes code simpler and sometimes fully specified if statement makes the code more readable. It is up to designer to choose how to avoid latch.
In additional to always_comb , you can also explicitly create a latch inside always_ff , especially asynchronous reset is used:
In the example above we are missing the condition where rst_n is high. Since a ’s value change only depends on rst_n (asynchronous reset), a actually does not depends on the clock edge. Hence the synthesis tool will infer a latch, instead of a flip-flop. Then again, since we use always_ff , an error/warning will be issued from synthesis tools.
To avoid creating latch, besides being careful when writing the logic, we can also resort to commercial SystemVerilog linters or even an elaboration analysis from synthesis tools. We will not cover linter in this book.
3.4.1.3 if Statement with Reset Logic
Although allowed by the language specification, stacking two if statements in the always_ff is not allowed in some synthesis tools, such as Design Compiler ® :
The code above will trigger ELAB-302 error since it contains two if statements in the always_ff block. There are two solutions for that:
- If a and b are totally separate, use two always_ff instead.
- If a and b are related, e.g. sharing the same input conditions, merge these two if statements into one if statement.
3.4.2 case Statement
case statement is similar to switch statement in C/C++ with some semantic difference due to the nature of hardware design. The general syntax for case is shown below:
Notice that we do not have break statement inside each case condition clause, which is the major difference compared to that of C/C++. As a result, there is no switch fall through in SystemVerilog. To take into the intentional fall through use case (shown in C++ below), we can put multiple conditions in the same case statement.
If there is only one statement for a particular case condition, we can omit begin...end . However, if any conditions has more than one statement, we recommend to use begin...end to enclose all conditions for readability.
SystemVerilog also allows to use range and wildcards as conditions using inside keyword, which is shown below:
In the example, ? is regarded as don’t care , which means it will match with any 4-state bit. For instance, if a = 4'b0xxxx , it will match with the first case. [8:12] is a range construct that is lower and upper bound inclusive. For instance, if a = 4'b1001 , it will match with the second case. If nothing matches, it will go to the default condition.
Like if statement, we can add modifiers to case statement. The most commonly used is unique . Keep in mind that the default case statement has priority. That is, if two conditions overlap, the execution will follow the first condition lexically. The synthesis tools are required to obey this convention as well. To produce optimal circuit, physical design engineers often use synthesis directives to remove such priority. However, removing priority creates an inconsistency between the simulator and synthesis tools, resulting in potential bug that can only be caught during gate-level simulation. Using unique forces the simulator to check if there is any conditions overlapping, which guarantee the consistency among tools. However, although using unique is highly recommended whenever possible, in some large designs, we may see exponential growth in runtime with some tools, e.g. Formality® from Synopsys®.
3.4.3 Loop Statements
Like C/C++, SystemVerilog also offers for and while loop for control logic. However, since the synthesis tools need to compile the logic into logical gates that compute in finite and deterministic cycles, the loop-bound has to be known during compile time; otherwise the tool either reports an error, or generated unwanted logic.
The general syntax for for loop is shown below:
Here we use i as int since it doesn’t matter whether i is 2-state for 4-state values. In situation where index i is used in arithmetics with 4-logic values, we need to declare i as 4-state variable. Notice that the loop upper bound 42 is a static value known during compilation time. If we use a variable as upper bound, a latch will be used since the synthesis tool assumes the upper bound could be 0, in such case the value update follows the latch inferring rules. However, we can disregard such rules when using for loops in test bench code, since the simulator is less picky.
The general syntax for while loop is shown below:
Unlike for loop, most synthesis tools cannot take while loop construct, since that requires compile-time full elaboration of the loop body, which can be tricky to do. We can convert the loop body into a for loop if needed. Again, there is no such restriction in the test bench code.
There is a variant of while loop in SystemVerilog, i.e. do...while , which has similar semantics as C/C++. The syntax for do...while loop is
To exit the loop body early, we can use the break statement, similar to C/C++. Some synthesis tools will optimize the circuit when it notices the break statement.
3.5 Generate statements
SystemVerilog allows users to “dynamically” create circuit logic using generate construct. Users can use for-loops or conditional generation to meta-program the circuit. However, such meta-programming has limitations:
- Generate is evaluated during elaboration time. Hence we cannot add or remove circuit during runtime.
- We cannot create ports using generate; net, such as logic , is allowed.
3.5.1 Loop generate
The syntax for loop generate is shown below:
Notice that unlike normal for loop, we need to declare the loop variable i using keyword genvar . However, genvar is used as an integer during elaboration to evaluate generated constructs. The loop bounds has to be known during compilation elaboration time, otherwise an error will be thrown. Loop generate can also be nested together to create more complex circuit logics.
When creating instance using generate statement, the hierarchy name is slightly different. We will cover the naming convention in next section.
3.5.2 Conditional generate
In many cases we need to conditionally generate logic based on the parametrization. SystemVerilog supports conditional generate constructs, as long as they can be elaborated statically during compilation time. The “conditional” part is typically done via if or case statements where the condition expression value is known. For instance, we can do the following conditional generate:
In the example we create instances based on the value of the parameter value . When module GenMod is instantiated with different parameter values, we will create instances accordingly. We can also create variables and connecting them inside the generate statement. For instance
3.6 Named Blocks, Scope Rules, and Hierarchical Names
SystemVerilog offers couple language features that allow programmers debug their hardware design easier than before. One demand for reliable debugging is the ability to address every signal by name hierarchically. With that ability, we can add assertions or verification tasks to the signals of interest without changing the design. This feature is achieved by allowing named blocks and defining scope rules.
3.6.1 Named Blocks
In SystemVerilog, you can name any code blocks defined by begin ... end , which can be used later on for hierarchical data access. The general syntax for naming code blocks is shown below:
In the example above, we created two named blocks with the name name_1 and name_2 respectively. Notice that people also add the names next to the end keyword so that it would be symmetric to the name used next to begin , which is, again, a form of coding style.
Notice that there are several cases where named blocks are highly recommend since it helps you to debug the code:
- The code blocks are generated through generate construct. By language specification, if the generated code block is not named, it will obtain genblk{NUM} as its identifier, where {NNUM} is substituted with the index of generated code blocks in the module scope.
- In cases such as functions or always_comb where you declare a temporary variable inside the scope, it is always a good practice to name the block so that you can refer to it later when debugging with the waveform, or setting up assertions.
- Using labels will also help to reader to identify the scope. This particular helpful when the number of lines in the code block is more than 20 lines. This is particular true for functions and modules.
From now on we will use named block whenever appropriate.
3.6.2 Scope Rules
Similar to software programming languages, SystemVerilog has a set of scoping rules. The following constructs define a new scope:
- begin-end blocks (named or unnamed)
- fork-join blocks (named or unnamed)
- Generate blocks
We have covered most of the constructs so far and will cover the reset of it later in the book.
In general, variables created in outer scope can be accessed in an inner scope, and illegal access the other way around. Two scopes at the same levels are isolated as well. Although it is somewhat unrelated to the scope rules, variable declaration inside a begin-end blocks need to follow ANSI-C style, that is, variable declaration has to be at the beginning at the scope. Declaration with assignment counts as normal statement, hence is illegal in the middle of statements, as shown below:
3.6.3 Hierarchical Names
In SystemVerilog, every identifier has a unique hierarchical path name . To do so, we can use “dot-notation” where . is used to access the child scope. There are some rules when resolving hierarchical names:
- You can always access child scope variables.
- If the parent scope is visible to the child scope, the child scope can use parent scope’s identify to access other scopes/variables.
Here is an example of accessing hierarchical names:
In general all the identifiers are public in a scope, meaning you are always be able to access it hierarchically. One exception is class variables declared as local , which will be covered later in the book.
3.6.4 Lifetime
Variables declared inside a module and interface have a static life lifetime by default. That means that all the variable will be instantiated/mapped at the beginning of simulation time, and will remain the same through out the entire simulation time. Variables declared inside a function or task by default are static. That means if a function is called multiple times without finishing, e.g. recursive calls or called through fork , the static variables will be overridden unexpectedly. To solve this issue, we can either declare the function/task automatic , e.g. function automatic foo() , or declare the variable automatic, e.g. automatic logic var . All variables declared inside an automatic function/task is by default non-static, as their lifetime is set to the life-time of the parent scope, which is similar to local variable declared inside a function in C/C++. Notice that automatic works with synthesizable code as well, even though the semantics are defined in terms of simulation environment.
3.7 Interface
Interface is a new construct introduced by SystemVerilog to encapsulate the reusable communication between different entities such as design and verification blocks. The concept of “interface” is similar to that of software programming language such as C#. However, instead of providing public accessible functions, interface in SystemVerilog defines a bundle of port names, connections, and functions associated with the ports.
Interface can be used as ports or internal wires that connects instances. Below is an example of configuration bus interface and its instantiation and usage. Notice that if the interface does not have any input/output ports, we need to instantiate it with () . Module config_reg takes a “generic” type port using the keyword interface . As a result, the module can take any be instantiated with any interface wires as long as it has required definitions. In other words, the config bus is abstracted away at the config_reg level, a concept similar to object-oriented programming’s generic concept. The legality will be check at compile time as usual.
You can also explicitly specify the interface type in the module definition, as shown below:
Below is an example of interface that has input and output ports. Notice that the instantiate is similar to that of module.
SystemVerilog also allows using keyword modport to create a “new interface” within the interface by specifying the directions of wires within the scope of the interface, as shown in the example below.
Because modport allows us to specify wire direction, the compiler can check the connections and make sure there is no multiple drivers on the same wire. By default, any wire declared inside the interface as inout connectivity. As a result, some synthesis tools may give warnings even though the connections is correct, hence modport is recommended. Since the wires are all connected together, we don’t need to explicit wire the connection inside the interface, i.e. master.clk is the same signal as slave.clk . modport construct can be used as any other interface when connected to module instances. Again, you can also specify the interface type in the module definition as below:
Like module definitions, interface in SystemVerilog also supports parametrization. The syntax is identical to that of modules, as shown below.
You can also add functions and tasks to the interface. Notice that for synthesizable RTL code we are limited to functions or tasks without any timing control logic. In addition, the function also has to be declared as automatic . Below is an example about interface with functions.
Continuous Assignment and Combinational Logic in SystemVerilog
In this post, we primarily talk about the concept of continuous assignment in SystemVerilog . We also look at how we can use this in conjunction with the SystemVerilog operators to model basic combinational logic circuits .
However, continuous assignment is a feature which is entirely inherited from verilog so anyone who is already familiar with verilog can skip this post.
There are two main classes of digital circuit which we can model in SystemVerilog – combinational and sequential .
Combinational logic is the simplest of the two, consisting solely of basic logic gates, such as ANDs, ORs and NOTs. When the circuit input changes, the output changes almost immediately (there is a small delay as signals propagate through the circuit).
In contrast, sequential circuits use a clock and require storage elements such as flip flops . As a result, output changes are synchronized to the circuit clock and are not immediate.
In the rest of this post, we talk about the main techniques we can use to design combinational logic circuits in SystemVerilog.
In the next post, we will discuss the techniques we use to model basic sequential circuits .
Continuous Assignment in SystemVerilog
In verilog based designs, we use continuous assignment to drive data on verilog net types . As a result of this, we use continuous assignment to model combinational logic circuits.
In SystemVerilog, we often use the logic data type rather than the verilog net or reg types. This is because the behavior of the logic type is generally more intuitive than the reg and wire types.
Despite this, we still make use of continuous assignment in SystemVerilog as it provides a convenient way of modelling combinational logic circuits.
We can use continuous assignment with either the logic type or with net types such as wire.
In SystemVerilog, we can actually use two different methods to implement continuous assignment.
The first of these is known as explicit continuous assignment. This is the most commonly used method for continuous assignment in SystemVerilog.
In addition, we can also use implicit continuous assignment, or net declaration assignment as it is also known. This method is less common but it can allow us to write less code.
Let's look at both of these techniques in more detail.
- Explicit Continuous Assignment
We normally use the assign keyword when we want to use continuous assignment in SystemVerilog. This approach is known as explicit continuous assignment.
The SystemVerilog code below shows the general syntax for continuous assignment using the assign keyword.
In this construct, we use the <variable> field to give the name of the signal which we are assigning data to. As we mentioned earlier, we can only use continuous assignment to assign data to net or logic type variables.
The <value> field can be a fixed value or we can create an expression using the SystemVerilog operators we discussed in a previous post.
When we use continuous assignment, the <variable> value changes whenever one of the signals in the <value> field changes state.
The code snippet below shows the most basic example of continuous assignment in SystemVerilog. In this case, whenever the b signal changes states, the value of a is updated so that it is equal to b.
- Net Declaration Assignment
We can also use implicit continuous assignment in our SystemVerilog designs. This approach is also commonly known as net declaration assignment in SystemVerilog.
When we use net declaration assignment, we place a continuous assignment in the statement which declares our signal. This can allow us to reduce the amount of code we have to write.
To use net declaration assignment in SystemVerilog, we use the = symbol to assign a value to a signal when we declare it.
The code snippet below shows the general syntax we use for net declaration assignment.
The variable and value fields have the same function for both explicit continuous assignment and net declaration assignment.
As an example, the SystemVerilog code below shows how we would use net declaration assignment to assign the value of b to signal a.
Modelling Combinational Logic Circuits in SystemVerilog
We use continuous assignment and the SystemVerilog operators to model basic combinational logic circuits in SystemVerilog.
In order to show we would do this, let's look at the very basic example of a three input and gate as shown below.
In order to model this circuit in SystemVerilog, we must use the assign keyword to drive the data on to the and_out output.
We can then use the bit wise and operator (&) to model the behavior of the and gate.
The code snippet below shows how we would model this three input and gate in SystemVerilog.
This example shows how simple it is to design basic combinational logic circuits in SystemVerilog. If we need to change the functionality of the logic gate, we can simply use a different SystemVerilog bit wise operator .
If we need to build a more complex combinational logic circuit, it is also possible for us to use a mixture of different bit wise operators.
To demonstrate this, let's consider the basic circuit shown below as an example.
In order to model this circuit in SystemVerilog, we need to use a mixture of the bit wise and (&) and or (|) operators. The code snippet below shows how we would implement this circuit in SystemVerilog.
Again, this code is relatively straight forward to understand as it makes use of the SystemVerilog bit wise operators which we discussed in the last post.
However, we need to make sure that we use brackets to model more complex logic circuit. Not only does this ensure that the circuit operates properly, it also makes our code easier to read and maintain.
Modelling Multiplexors in SystemVerilog
Multiplexors are another component which are commonly used in combinational logic circuits.
In SystemVerilog, there are a number of ways we can model these components.
One of these methods uses a construct known as an always block which we will discuss in detail in the next post. Therefore, we will not discuss this approach to modelling multiplexors in this post.
However, we will look at the other methods we can use to model multiplexors in the rest of this post.
- SystemVerilog Conditional Operator
As we talked about in a previous post, there is a conditional operator in SystemVerilog . This functions in the same way as the conditional operator in the C programming language.
To use the conditional operator, we write a logical expression before the ? operator which is then evaluated to see if it is true or false.
The output is assigned to one of two values depending on whether the expression is true or false.
The SystemVerilog code below shows the general syntax which the conditional operator uses.
From this example, it is clear how we can create a basic two to one multiplexor using this operator.
However, let's look at the example of a simple 2 to 1 multiplexor as shown in the circuit diagram below.
The code snippet below shows how we would use the conditional operator to model this multiplexor in SystemVerilog.
- Nested Conditional Operators
Although this is not common, we can also write code to build larger multiplexors by nesting conditional operators.
To show how this is done, let's consider a basic 4 to 1 multiplexor as shown in the circuit below.
In order to model this in SystemVerilog using the conditional operator, we treat the multiplexor circuit as if it were a pair of two input multiplexors.
This means one multiplexor will select between inputs A and B whilst the other selects between C and D. Both of these multiplexors use the LSB of the address signal as the address pin.
The SystemVerilog code shown below demonstrates how we would implement this.
To create the full four input multiplexor, we would then need another multiplexor.
This multiplexor then takes the output of the other two multiplexors and uses the MSB of the address signal to select between.
The code snippet below shows the simplest way to do this. This code uses the signals mux1 and mux2 which we defined in the last example.
However, we could easily remove the mux1 and mux2 signals from this code and instead use nested conditional operators.
This reduces the amount of code that we would have to write without affecting the functionality.
The code snippet below shows how we would do this.
As we can see from this example, when we use conditional operators to model multiplexors in verilog, the code can quickly become difficult to understand. Therefore, we should only use this method to model small multiplexors.
- Arrays as Multiplexors
It is also possible for us to use basic SystemVerilog arrays to build simple multiplexors.
In order to do this, we combine all of the multiplexor inputs into a single array type and use the address to point at an element in the array.
In order to get a better idea of how this works in practise, let's consider a basic four to one multiplexor as an example.
The first thing we must do is combine our input signals into an array. There are two ways in which we can do this.
Firstly, we can declare an array and then assign all of the individual bits, as shown in the SystemVerilog code below.
Alternatively we can use the SystemVerilog concatenation operator , which allows us to assign the entire array in one line of code.
In order to do this, we use a pair of curly braces - { } - and list the elements we wish to include in the array inside of them.
When we use the concatenation operator we can also declare and assign the variable in one statement.
The SystemVerilog code below shows how we can use the concatenation operator to populate an array.
As SystemVerilog is a loosely typed language , we can use the two bit addr signal as if it were an integer type. This signal then acts as a pointer that determines which of the four elements to select.
The code snippet below demonstrates this method in practise.
What is the difference between implicit and explicit continuous assignment?
When we use implicit continuous assignment we assign the variable a value when we declare. In contrast, when we use explicit continuous assignment we use the assign keyword to assign a value.
Write the code for a 2 to 1 multiplexor using any of the methods discussed in this post.
Write the code for circuit below using both implicit and explicit continuous assignment.
Leave a Reply Cancel reply
Your email address will not be published. Required fields are marked *
Save my name, email, and website in this browser for the next time I comment.
Table of Contents
Sign up free for exclusive content.
Don't Miss Out
We are about to launch exclusive video content. Sign up to hear about it first.
Verilog Conditional Statements
In Verilog, conditional statements are used to control the flow of execution based on certain conditions. There are several types of conditional statements in Verilog listed below.
Conditional Operator
The conditional operator allows you to assign a value to a variable based on a condition. If the condition is true, expression_1 is assigned to the variable. Otherwise, expression_2 is assigned.
Nested conditional operators
Conditional operators can be nested to any level but it can affect readability of code.
Here are some of the advantages of using conditional operators:
- Concise syntax : The conditional operator allows for a compact and concise representation of conditional assignments. It reduces the amount of code needed compared to using if-else statements or case statements.
- Readability : The conditional operator can enhance code readability, especially for simple conditional assignments. It clearly expresses the intent of assigning different values based on a condition in a single line.
And some disadvantages:
- Limited functionality : The conditional operator is primarily used for simple conditional assignments. It may not be suitable for complex conditions or multiple actions, as it can quickly become unreadable and difficult to maintain.
- Lack of flexibility : The conditional operator only allows for a binary choice based on the condition. It cannot handle multiple cases or multiple actions within a single line of code.
- Potential for reduced readability : While the conditional operator can enhance code readability for simple assignments, it can also make the code more difficult to understand if the condition and assigned values become complex.
if-else statement
The if-else statement allows you to perform different actions based on a condition.
If the condition evaluates to true, statement 1 is executed. Otherwise, statement 2 is executed.
Read more on Verilog if-else-if statements
case statement
The case statement is used when you have multiple conditions and want to perform different actions based on the value of a variable.
The expression is evaluated, and based on its value, the corresponding statement is executed. If none of the values match the expression, the statement under default is executed.
Read more on Verilog case statement
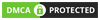
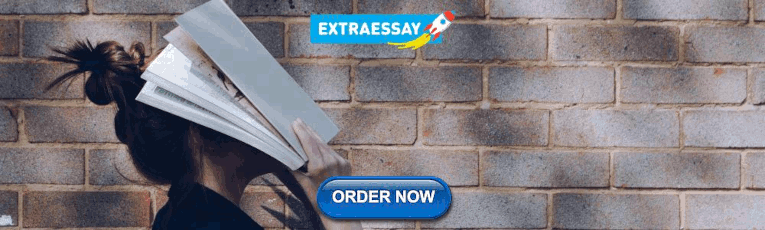
IMAGES
VIDEO
COMMENTS
The LHS can be a bit-select of a net, part-select of a net, variable or a net but cannot be the reference to an array and bit/part select of a variable. The force statment will override all other assignments made to the variable until it is released using the release keyword. reg o, a, b; initial begin force o = a & b; ... release o; end
var_b = 0100. ncsim: *W,RNQUIE: Simulation is complete. In the previous article, an overview of the major data types were given. In this session, we'll look at 4-state and 2-state variables and two new data types called logic and bit. 4-state data types Types that can have unknown (X) and high-impedance (Z) value in addition to zero (0) and one ...
The choice of the name reg turned out to be a mistake, because the existence of registers is instead inferred based on how assignments are performed.Due to this, use of reg is essentially deprecated in favor of logic, which is actually the same type.. logic is a 1-bit, 4-state data type. bit is a 1-bit, 2-state data type which may simulate faster than logic
Verilog assign statement. Signals of type wire or a similar wire like data type requires the continuous assignment of a value. For example, consider an electrical wire used to connect pieces on a breadboard. As long as the +5V battery is applied to one end of the wire, the component connected to the other end of the wire will get the required ...
Assignment Operators in System Verilog. In System Verilog, we use assignment operators to assign values to variables. ... The bit-select operator [] and the part-select operator [start:end] are used to select a single bit or a range of bits from a vector, respectively. The syntax for the bit-select operator is vector[index], where vector is the ...
It's part of SystemVerilog's move towards unifying the data type system. A logic in SystemVerilog is essentially a 4-state data type. The four states are 0, 1, X, and Z. In terms of declaration and usage, logic acts just like reg type. It can hold a value and you can write procedural assignments to it, similar to variables in other languages:
Typical Verilog arrays are fixed length at compile time bit [3:0] arr [3]; // a 3 element array of 4 bit values arr = '{12, 10, 3}; // a literal array assignment Dynamic arrays are sized at runtime Useful for generating variable length stimulus bit [3:0] arr []; // a dynamic array of 4 bit values initial begin
language took on the name SystemVerilog, and was released in 2009. At this point Verilog was ... module two_bit_mux (inputlogic [1:0] a, b, inputlogic sel, outputlogic [1:0] f); ... This is not a one-time assignment but a continuous one. Note that continuous assignments, like
SystemVerilog for Verification Overview New Data Types Dynamic Arrays bit [ 3: 0] arr [ 3]; // a 3 element array of 4 bit values arr = ' { 12, 10, 3}; // a literal array assignment bit 3 0 initial begin
Looking for an elegant way I can define range of bits I am accessing in a vector, in some common place, which I can modify it and it will be waterfalled to all files in code using it, for example: expected_txn.raw_data [rgb_pos-:5] = data_range_bus [28:24]; expected_txn.raw_data [rgb_pos+6-:6] = data_range_bus [17:12]; Will be happy if it could ...
Packed data always goes from MSB to LSB in field declaration order. If you declare. mem_pkt pkt; Then pkt.addr[7] corresponds to pkt[39] and pkt.addr[0] corresponds to pkt[32].pkt.data[0] corresponds to pkt[0]. Verilog is a weakly typed language and there is no way prevent assignments from packed arrays and structs to one another without some kind of linting tool.
In SystemVerilog, Continuous Assignments are a fundamental way to model the behavior of digital circuits at a low level. They closely mirror how hardware systems function, where signals propagate through wires and gates spontaneously, without requiring a clock tick or control flow. Continuous Assignments are primarily associated with the wire ...
I would like to create a parametric bit-width assignment in Verilog. Something like the following code: module COUNTER ( CLEAR, ... Stack Exchange Network. Stack Exchange ... system-verilog; synthesis; Share. Cite. Follow edited Jan 23, 2023 at 15:01. toolic. 8,332 7 7 ...
The SystemVerilog byte type is an 8 bit data type which we can use to model whole numbers. By default, the byte type is is encoded as a signed 2s complement number. As a result of this, it can only accept values from -127 to 127. However, we can also declare the the byte type to be encoded as an unsigned number.
In the example shown below, a static array of 8-bit wide is declared, assigned some value and iterated over to print its value. bit [7:0] m_data; // A vector or 1D packed array. initial begin. // 1. Assign a value to the vector. m_data = 8'hA2; // 2. Iterate through each bit of the vector and print value.
Since cout is only 1-bit, it gets the value of carry out. ```SystemVerilog // a 4-bit adder with carry logic [3:0] a; logic [3:0] b; logic [3:0] sum; ... You cannot mix the bit assignment with other procedural blocks either. The evaluation of each statement is in-order. The simulator will go through each statement from top to bottom and ...
ASSIGNMENTS IN VERILOG. ... Bit-select of reg, integer or time. Part-select of reg, ... SYSTEM VERILOG STATIC AND AUTOMATIC LIFETIME OF VARIABLE AND METHODS. Static: For a variable static lifetime ...
This approach is known as explicit continuous assignment. The SystemVerilog code below shows the general syntax for continuous assignment using the assign keyword. assign <variable> = <value>; In this construct, we use the <variable> field to give the name of the signal which we are assigning data to.
Typical Verilog arrays are fixed length at compile time bit [ 3: 0] arr [ 3]; // a 3 element array of 4 bit values arr = ' { 12, 10, 3}; // a literal array assignment Dynamic arrays are sized at runtime Useful for generating variable length stimulus bit [ 3: 0] arr []; // a dynami c array of 4 bi t val ues initial begin
I have the following SystemVerilog variable: bit [5:0] my_bits = 6'h3E; // my_bits == 6'd62 ... The explicit int'() cast is unnecessary since the assignment to an int variable creates an implicit cast to int. Also realize you are taking a chance with these variable declaration initializations as the order they are performed is undefined ...
In Verilog, conditional statements are used to control the flow of execution based on certain conditions. There are several types of conditional statements in Verilog listed below. Conditional Operator <variable> = <condition> ? <expression_1> : <expression_2>; The conditional operator allows you to assign a value to a variable based on a ...