
U.S. Government Accountability Office
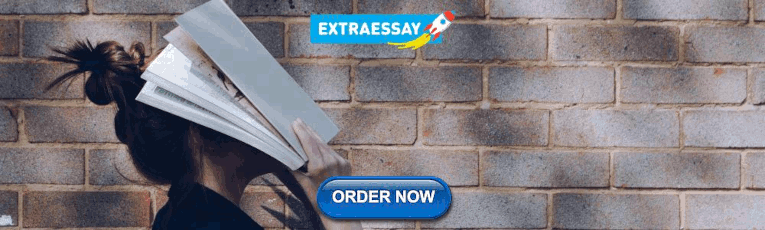
Earthquakes: Opportunities Exist to Further Assess Risk, Build Resilience, and Communicate Research
The National Earthquake Hazards Reduction Program helps U.S. communities strengthen their earthquake resilience. For example, the program educates the public on earthquake risks and helps communities update building codes and improve design and construction practices.
The program has started assessing progress, for example, by tracking building code adoption in 22,000 jurisdictions. But we found that a national assessment hasn't been done. Such an assessment could help the program more strategically address inconsistencies in how states, localities, territories, and tribes mitigate earthquake risks.
Our recommendations address this, and more.
Damage from the 2020 Southwest Puerto Rico Earthquake Sequence
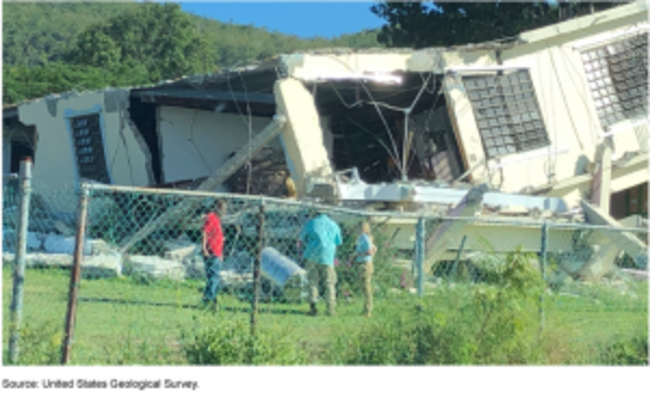
What GAO Found
The National Earthquake Hazards Reduction Program (NEHRP) has goals outlined in its most recent Strategic Plan for fiscal years (FY) 2009-2013 for the improvement of earthquake resilience in communities nationwide. However, officials from the National Institute of Science and Technology (NIST) said that a national risk assessment has not been done to identify improvements and remaining gaps in resilience. The Federal Emergency Management Agency (FEMA) initiated some efforts to identify improvements by collecting data on the adoption of building codes. The NEHRP agencies are currently working to update the Strategic Plan FY 2022-2029. By conducting a national risk assessment, NEHRP would gain greater awareness of earthquake resilience improvements and be better positioned in planning long-term goals and objectives toward closing remaining gaps.
Damage to Anchorage, Alaska Following a Magnitude 7.1 Earthquake in 2018
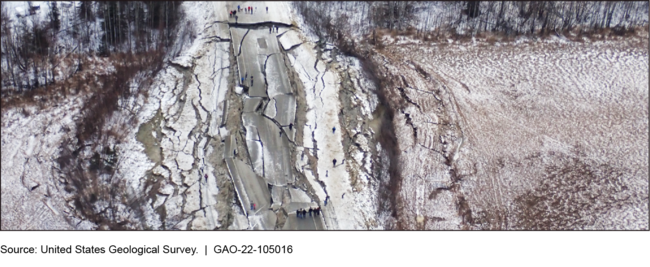
Accomplishing NEHRP's strategic objectives requires developing and applying research in the geological, engineering, and social sciences areas. NEHRP identifies research priorities, and many of the NEHRP agencies award grants to entities such as universities or state and local agencies, to conduct research. While the communication mechanisms used by the National Science Foundation (NSF) include program solicitations, program descriptions, and letters issued to research entities, they do not communicate NEHRP's strategic research priorities. By developing strategies to better communicate its research priorities, NEHRP can help ensure that they are met.
NEHRP's Program Coordination Working Group is responsible for coordinating the implementation of NEHRP's strategic research priorities and has followed leading practices for leadership and outcomes. However, the working group did not follow two leading practices for accountability and resources. For example, the working group did not track and monitor progress, and did not identify and leverage resources needed to achieve outcomes for research priorities. Identifying resources would enable the interagency group to leverage all relevant resources across the NEHRP agencies, and better align them with research priorities. Further, the identification of resources would provide an opportunity for the working group to build programmatic partnerships aimed at strengthening earthquake resilience.
Why GAO Did This Study
Established in 1977, NEHRP aims to help reduce the risks to life and property from earthquakes. NEHRP's initiatives include strengthening community resilience through improved design and construction methods, conducting research to better understand the impacts from earthquakes, and providing outreach and education. NEHRP is comprised of four federal agencies (FEMA, NIST, NSF, and the U.S. Geological Survey) that promote and support NEHRP's initiatives for strengthening earthquake resilience.
The National Earthquake Hazards Reduction Program Reauthorization Act of 2018 includes a provision for GAO to assess the program's efforts. This report examines, among other things, NEHRP's progress in identifying gaps and strengthening resilience to earthquakes, and its activities to identify and communicate about research priorities. GAO reviewed NEHRP's strategic plans, agency guidance, and external communications; compared procedures to leading practices for interagency collaboration; and interviewed federal and state officials, among others.
Recommendations
GAO is making seven recommendations, including that NEHRP agencies conduct a national assessment to identify progress and remaining gaps in earthquake resilience; develop strategies to better communicate research priorities; and follow leading practices to identify and leverage resources. NIST, NSF, and FEMA concurred with our recommendations.
Recommendations for Executive Action
Full report, gao contacts.
Christopher P. Currie Director [email protected] (404) 679-1875
Office of Public Affairs
Chuck Young Managing Director [email protected] (202) 512-4800
Thank you for visiting nature.com. You are using a browser version with limited support for CSS. To obtain the best experience, we recommend you use a more up to date browser (or turn off compatibility mode in Internet Explorer). In the meantime, to ensure continued support, we are displaying the site without styles and JavaScript.
- View all journals
- My Account Login
- Explore content
- About the journal
- Publish with us
- Sign up for alerts
- Open access
- Published: 06 August 2021
Machine learning and earthquake forecasting—next steps
- Gregory C. Beroza 1 ,
- Margarita Segou 2 &
- S. Mostafa Mousavi 1
Nature Communications volume 12 , Article number: 4761 ( 2021 ) Cite this article
20k Accesses
59 Citations
48 Altmetric
Metrics details
- Natural hazards
A new generation of earthquake catalogs developed through supervised machine-learning illuminates earthquake activity with unprecedented detail. Application of unsupervised machine learning to analyze the more complete expression of seismicity in these catalogs may be the fastest route to improving earthquake forecasting.
The past 5 years have seen a rapidly accelerating effort in applying machine learning to seismological problems. The serial components of earthquake monitoring workflows include: detection, arrival time measurement, phase association, location, and characterization. All of these tasks have seen rapid progress due to effective implementation of machine-learning approaches. They have proven opportune targets for machine learning in seismology mainly due to the large, labeled data sets, which are often publicly available, and that were constructed through decades of dedicated work by skilled analysts. These are the essential ingredient for building complex supervised models. Progress has been realized in research mode to analyze the details of seismicity well after the earthquakes being studied have occurred, and machine-learning techniques are poised to be implemented in operational mode for real-time monitoring. We will soon have a next generation of earthquake catalogs that contain much more information. How much more? These more complete catalogs typically feature at least a factor of ten more earthquakes (Fig. 1 ) and provide a higher-resolution picture of seismically active faults.
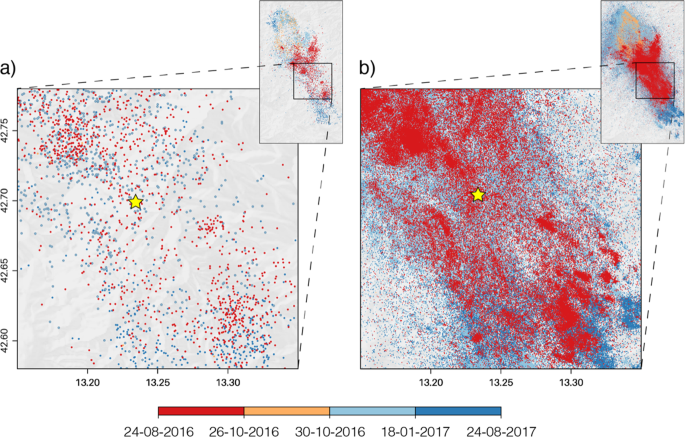
a Real-time catalog, available at http://cnt.rm.ingv.it/ and ( b ) machine-learning catalog 16 are shown for event magnitudes above their respective magnitude of completeness 12 , 16 Mc = 2.2 and Mc = 0.5.
This next generation of earthquake catalogs will not be the single, static objects seismologists are accustomed to working with. For example, less than 2 years after the 2019 Ridgecrest, California earthquake sequence there already exist four next-generation catalogs, each of which were developed with different enhanced detection techniques. Now, and in the future, this will be the norm, and earthquake catalogs will be updated and improved—potentially dramatically—with time. Second-generation deep learning models 1 that are specifically designed based on earthquake signal characteristics and that mimic the manual processing by analysts, can lead to performance increases beyond those offered by earlier models that adapted neural network architectures from other fields. Those interested in using earthquake catalogs for forecasting can anticipate a shifting landscape with continuing improvements.
While these improvements are impressive, the value of the extra information they provide is less clear. What will we learn about earthquake behavior from these deeper catalogs and how might it improve the prospects for the stubbornly difficult problem of earthquake forecasting?
Short-term deterministic earthquake prediction remains elusive and is perhaps impossible; however, probabilistic earthquake forecasting is another matter. It remains the subject of focused and sustained attention and it informs earthquake hazard characterization 2 and thus both policy and earthquake risk reduction. A key assumption is that what we learn from the newly uncovered small earthquakes in AI-based catalogs, will inform earthquake forecasting for events of all magnitudes. The observed scale invariance of earthquake behavior suggests this is a reasonable expectation.
Empirical seismological relationships have played a key role in the development of earthquake forecasting. These include Omori’s law 3 that describes the temporal decay of aftershock rate, the magnitude-frequency distribution, with the b-value describing the relative numbers of small vs. large earthquakes 4 , and the Epidemic Type Aftershock Sequence (ETAS) model 5 in which earthquakes are treated as a self-exciting process governed by Omori’s law for their frequency of occurrence and Gutenberg–Richter statistics for their magnitude. These empirical laws continue to prove their utility. Just in the past few years, the time dependence of the b-value has been used to try to anticipate the likelihood of large earthquakes during an ongoing earthquake sequence 6 and the ETAS model has been improved to better anticipate future large events 7 . So it appears that there is value in applying these longstanding relationships to improved earthquake catalogs, but our opinion is that much more needs to be done.
The relationships cited above date from 127, 77, and 33 years ago. The oldest of them, Omori’s Law, was developed based on felt reports without the benefit of instrumental measurements. We suggest that a fresh approach using more powerful techniques is warranted. Earthquake catalogs are complex, high-dimensional objects and as Fig. 1 makes clear, that is even more true for the deeper catalogs that are being developed through machine learning. Their high dimensionality makes them challenging for seismologists to explore, and the conventional approaches noted above seem unlikely to be taking advantage of the wealth of new information available in the new generation of deeper catalogs. We suggest that, having first enabled the development of these catalogs, the statistical-learning techniques of data science are now poised to play an important role in uncovering new relationships within them. The obvious next step is to apply the techniques of machine learning in discovery mode 8 to discern new relationships encoded in the seismicity.
There are tantalizing indications that such an approach may lead to new insights. In double-direct-shear experiments, background signals that were thought to be uninformative random noise have instead been shown to encode information on the state of friction and the eventual time of failure of faults in a laboratory setting 9 . Well-controlled laboratory analogs to faults lack the geologic complexity of the Earth, yet, weak natural background vibrations of a similar sort, that again were thought to be random noise, have been shown to embody information that can be used to predict the onset time of slow slip events in the Cascadia subduction zone 10 . Finally, unsupervised deep learning, in which algorithms are used to discern patterns in data without the benefit of prior labels, applied to seismic waveform data uncovered precursory signals preceding the large and damaging 2017 landslide and tsunami in Greenland 11 .
These examples are compelling but come with the caveat that they are not representative of the typical fast rupture velocity earthquakes on tectonic faults that are of societal concern. For such earthquakes, however, there are also indications from state-of-the-art forecasting approaches that next-generation earthquake catalogs may contain information that will lead to progress. Physics-based forecasting models, which account for changes in the Coulomb failure stress due to antecedent earthquakes that favor the occurrence of subsequent earthquakes, have shown increasing skill such that they are competitive with, and are beginning to outperform, statistical models. Coulomb failure models benefit particularly from deeper catalogs because they include many more small magnitude earthquakes. These small earthquakes add predictive power through their secondary triggering effects tracking the evolution of the fine-scale stress field that ultimately controls earthquake nucleation in foreshock and aftershock sequences. They can also be used to define the emerging active structures that comprise fault networks and by doing so clarify the relevant components of stress that would act to trigger earthquakes 12 . Secondary triggering and background stress heterogeneity were shown to improve stress triggering models 13 but were most effective when they incorporated near‐real‐time aftershock data from the sequence as it unfolded 14 . We note that there is no reason why more complete earthquake catalogs, developed with pre-trained neural network models, cannot be created in real time as an earthquake sequence unfolds. Finally, despite the disappointing history of the search for precursors, due diligence requires that seismologists consider the pursuit of signals that might be precursory.
We conclude that it is now possible to image the activity on active fault systems with unprecedented spatial resolution. This will enable experimentation with familiar hypotheses and enable the formulation of new hypotheses. It seems certain that the underlying processes that drive earthquake occurrence are encoded in this next generation of earthquake catalogs, but we may not find them unless we put new effort into searching for them. Unsupervised learning methods 15 are particularly well-suited tool for that effort.
Mousavi, S. M. et al. Earthquake Transformer - an attentive deep-learning model for simultaneous earthquake detection and phase picking. Nat. Commun. 11 , 3952, https://doi.org/10.1038/s41467-020-17591-w (2020).
Field, E. H. et al. A synoptic view of the third Uniform California Earthquake Rupture Forecast model. Seismological Res. Lett. 88 , 1259–1267, https://doi.org/10.1785/0220170045 (2017).
Omori, F. J. On the aftershocks of earthquakes. Coll. Sci. Imp. Univ. Tokyo 7 , 111–200 (1894).
Google Scholar
Gutenberg, B. & Richter, C. F. Frequency of earthquakes in California. Bull. Seismol. Soc. Am. 34 , 185–188 (1944).
Article Google Scholar
Ogata, Y. Statistical models for earthquake occurrences and residual analysis for point processes. J. Am. Stat. Assoc. 83 , 9–27 (1988).
Gulia, L. & Wiemer, S. Real-time discrimination of earthquake foreshocks and aftershocks. Nature 574 , 193–199, https://doi.org/10.1038/s41586-019-1606-4 (2019).
Shcherbakov, R. et al. Forecasting the magnitude of the largest expected earthquake. Nat. Commun. 10 , 4051, https://doi.org/10.1038/s41467-019-11958-4 (2019).
Bergen, K. J. et al. Machine learning for data-driven discovery in solid earth geoscience. Science 363 , eaau0323, https://doi.org/10.1126/science.aau0323 (2019).
Article CAS PubMed Google Scholar
Rouet-Leduc, B. et al. Machine learning predicts laboratory earthquakes. Geophys. Res. Lett . 44 , 9276–9282 https://doi.org/10.1002/2017GL074677 (2017).
Hulbert, C. et al. An exponential build-up in seismic energy suggests a months-long nucleation of slow slip in Cascadia. Nat. Commun. 11 , 4139, https://doi.org/10.1038/s41467-020-17754-9 (2020).
Article ADS CAS PubMed PubMed Central Google Scholar
Seydoux, L. et al. Clustering earthquake signals and background noises in continuous seismic data with unsupervised deep learning. Nat. Commun. 11 , 3972, https://doi.org/10.1038/s41467-020-17841-x (2020).
Mancini, S. et al. Improving physics-based aftershock forecasts during the 2016-2017 Central Italy earthquake cascade. J. Geophys. Res. Solid Earth 124 , https://doi.org/10.1029/2019JB017874 (2019).
Segou, M., & Parsons, T. A new technique to calculate earthquake stress transfer and to probe the physics of aftershocks. Bull. Seismol. Soc. Am . 110 , 863–873, https://doi.org/10.1785/0120190033 (2020).
Mancini, S. et al. The predictive skills of elastic Coulomb rate-and-state aftershock forecasts during the 2019 Ridgecrest, California, earthquake sequence. Bull. Seismol. Soc. Am. 110 , 1736–1751, https://doi.org/10.1785/0120200028 (2020).
Mousavi, S. M., et al. Unsupervised clustering of seismic signals using deep convolutional autoencoders. IEEE Trans. Geosci. Remote Sens. Lett. 16 , 1693–1697, https://doi.org/10.1109/LGRS.2019.2909218 (2019).
Article ADS Google Scholar
Tan, Y. J. et al. Machine-learning-based high-resolution catalog reveals how complex fault structures were activated during the 2016-2017 Central Italy sequence. Seismic Rec. 1 , 11–19, https://doi.org/10.1785/0320210001 (2021).
Download references
Acknowledgements
This work is supported by the NERC-NSFGEO 13 funded project The Central Apennines Earthquake Sequence under a New Microscrope (NE/R0000794/1). G.C.B. was supported by Department of Energy (Basic Energy Sciences; Award DE-SC0020445). Thanks to Dr. Simone Mancini for preparing the figure.
Author information
Authors and affiliations.
Department of Geophysics, Stanford University, Stanford, CA, USA
Gregory C. Beroza & S. Mostafa Mousavi
British Geological Survey, Research Avenue South, Lyell Centre, Edinburgh, UK
Margarita Segou
You can also search for this author in PubMed Google Scholar
Contributions
All authors contributed to the development of this comment, and all were involved in revising it.
Corresponding author
Correspondence to Gregory C. Beroza .
Ethics declarations
Competing interests.
The authors declare no competing interests.
Additional information
Publisher’s note Springer Nature remains neutral with regard to jurisdictional claims in published maps and institutional affiliations.
Rights and permissions
Open Access This article is licensed under a Creative Commons Attribution 4.0 International License, which permits use, sharing, adaptation, distribution and reproduction in any medium or format, as long as you give appropriate credit to the original author(s) and the source, provide a link to the Creative Commons license, and indicate if changes were made. The images or other third party material in this article are included in the article’s Creative Commons license, unless indicated otherwise in a credit line to the material. If material is not included in the article’s Creative Commons license and your intended use is not permitted by statutory regulation or exceeds the permitted use, you will need to obtain permission directly from the copyright holder. To view a copy of this license, visit http://creativecommons.org/licenses/by/4.0/ .
Reprints and permissions
About this article
Cite this article.
Beroza, G.C., Segou, M. & Mostafa Mousavi, S. Machine learning and earthquake forecasting—next steps. Nat Commun 12 , 4761 (2021). https://doi.org/10.1038/s41467-021-24952-6
Download citation
Received : 28 May 2021
Accepted : 12 July 2021
Published : 06 August 2021
DOI : https://doi.org/10.1038/s41467-021-24952-6
Share this article
Anyone you share the following link with will be able to read this content:
Sorry, a shareable link is not currently available for this article.
Provided by the Springer Nature SharedIt content-sharing initiative
This article is cited by
An all-in-one seismic phase picking, location, and association network for multi-task multi-station earthquake monitoring.
Communications Earth & Environment (2024)
Deep learning forecasting of large induced earthquakes via precursory signals
- Vincenzo Convertito
- Fabio Giampaolo
- Francesco Piccialli
Scientific Reports (2024)
Cascade and pre-slip models oversimplify the complexity of earthquake preparation in nature
- Patricia Martínez-Garzón
The Role of Machine Learning in Earthquake Seismology: A Review
- Anup Chitkeshwar
Archives of Computational Methods in Engineering (2024)
Machine learning-based multipath modeling in spatial domain applied to GNSS short baseline processing
- Yuanxin Pan
- Gregor Möller
- Benedikt Soja
GPS Solutions (2024)
Quick links
- Explore articles by subject
- Guide to authors
- Editorial policies
Sign up for the Nature Briefing newsletter — what matters in science, free to your inbox daily.

Suggestions or feedback?
MIT News | Massachusetts Institute of Technology
- Machine learning
- Social justice
- Black holes
- Classes and programs
Departments
- Aeronautics and Astronautics
- Brain and Cognitive Sciences
- Architecture
- Political Science
- Mechanical Engineering
Centers, Labs, & Programs
- Abdul Latif Jameel Poverty Action Lab (J-PAL)
- Picower Institute for Learning and Memory
- Lincoln Laboratory
- School of Architecture + Planning
- School of Engineering
- School of Humanities, Arts, and Social Sciences
- Sloan School of Management
- School of Science
- MIT Schwarzman College of Computing
Shaking up earthquake research at MIT
Press contact :.
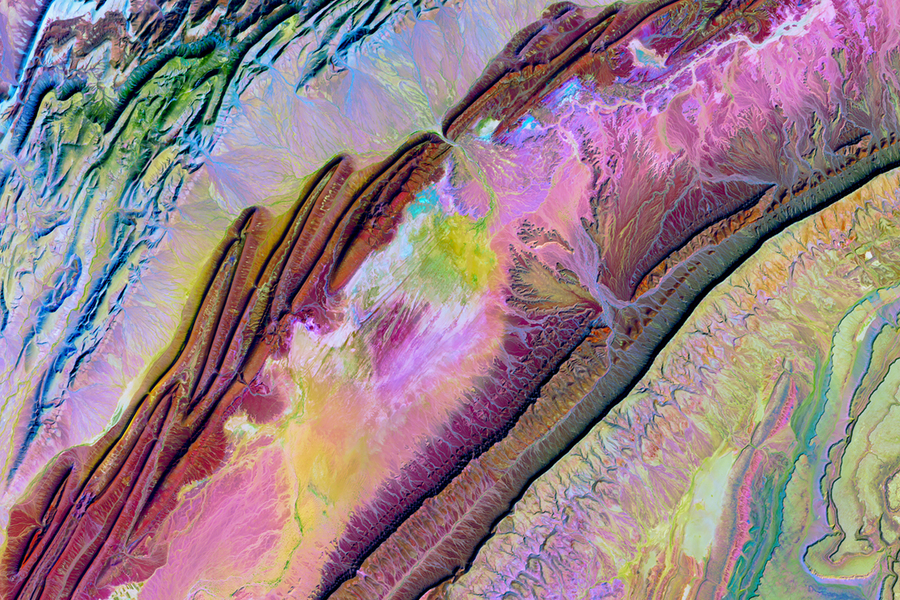
Previous image Next image
Major environmental events write their own headlines. With loss of life and crippling infrastructure damage, the aftershocks of earthquakes reverberate around the world — not only as seismic waves, but also in the photos and news stories that follow a major seismic event. So, it is no wonder that both scientists and the public are keen to understand the dynamics of faults and their hazard potential, with the ultimate goal of prediction.
To do this, William Frank and Camilla Cattania, assistant professors in MIT’s Department of Earth, Atmospheric and Planetary Sciences (EAPS), have teamed up as EQSci@MIT to uncover hidden earthquake behaviors and fault complexity, through observation, statistics, and modeling. Together, their complementary avenues of research are helping to expose the fault mechanics underpinning everything from aseismic events, like slow slip actions that occur over periods of hours or months, to large magnitude earthquakes that strike in seconds. They’re also looking at the ways tectonic regions interact with neighboring events to better understand how faults and seismic events evolve — and, in the process, shedding light on how frequently and predictably these events might occur.
“Basically, [we’re] trying to build together a pipeline from observations through modeling to answer the big-picture questions,” says Frank. “When we actually observe something, what does that mean for the big-picture result, in places where we have strong heterogeneity and lots of earthquake activity?”
Observing Earth as it creeps
While there are many ways to investigate different types of earthquakes and faults, Frank takes a detailed and steady approach: looking at slow-moving, low wave frequency earthquakes — called slow slip — in subduction zones over long periods of time. These events tend to go unnoticed by the public and lack an obvious seismic wave signature that would be registered by seismometers. However, they play a significant role in tectonic buildup and release of energy. “When we start to look at the size of these slow slip events, we realize that they are just as big as earthquakes,” says Frank.
His group leverages geodetic data, like GPS, to monitor how the ground moves on and near a fault to reveal what’s happening along the plate interface as you descend deeper underground. In the crust, near the surface, the plates tend to be locked together along the boundary, building up pressure and then releasing it as a giant earthquake. However, below that region, Frank says, the rocks are more elastic and can deform and creep, which can be picked up on instrumentation. “There are events that are transient. They happen over a set period of time, just like an earthquake, but instead of several seconds to minutes, they last several days to months,” he says.
Since slow slip has the capacity to cause energy loading in subduction zones through both stress and release, Frank and his group want to understand how slow earthquakes interact with seismic regions, where there’s potential for a large earthquake. By digging into observational data, from long-term readings to those taken on the scale of a few hours, Frank has learned that often there are many tiny earthquakes that repeat during slow slip. While a first glance at the data may look like just noise, clear signals emerge on closer inspection that reveal a lot about the subsurface plate interface — like the presence of trapped fluid, and how subduction zones behave at different locations along a fault.
“If we really want to understand where and when and how we're going to have a big earthquake, you have to understand what's happening around it,” says Frank, who has projects spread out around the globe, investigating subducting plate boundaries from Japan to the Pacific Northwest, and all the way to Antarctica.
Modeling complexity
Camilla Cattania’s work provides a counterpoint for Frank’s. Where the Frank group incorporates seismic and geodetic record collection, Cattania employs numerical, analytical, and statistical tools to understand the physics of earthquakes. Through modeling, her team can test hypotheses and then look for corroborating evidence in the field, or vice versa, using collected data to inform and refine models. Influenced by major seismic hazards in her home country of Italy, Cattania is keenly interested in the potential to contribute models for practical use in earthquake forecasting.
One aspect of her work has been to reconcile theoretical models with the complex reality of fault geometry. Each fault has its own physical characteristics that affect its behavior and can evolve over time — not just the dimensions of the fault, but also factors like the orientation of the rock fractures, the elastic properties of the rocks, and the irregularity and roughness of their surfaces. When looking into numerical models of aftershock sequences, she was able to show that they weren’t as predictive as statistical models because previous models were using idealized fault planes in the calculations.
To remedy this, Cattania explored ways to incorporate fault geometry that's more consistent with the complexity found in nature. “We were the first to implement this in a systematic way and then compare it to statistical models, and … to show that these physical models can do well, if you make them realistic enough,” she says.
Cattania has also been looking into modeling how the physical properties of faults control the frequency and size of earthquakes — a key question in understanding the hazards they pose. Some earthquake sequences tend to recur at intervals, but most don’t, defying easy prediction. In trying to understand why this is, Cattania explains, size is everything. “It turns out that periodicity is a property which depends on the size of the earthquake. It's much more unlikely to get periodic behavior for a large earthquake than it is for a small one, and it just comes out of the fundamental physics of how friction and elasticity control the cycle,” she says.
A synergistic approach
Ultimately, through their collaboration in EAPS at MIT, Frank and Cattania are trying to build more communication between observation and modeling in order to foster more rapid advancements in earthquake science. “Ever-improving seismic and geodetic measurements, together with new data analysis techniques, are providing unprecedented opportunities to probe fault behavior,” says Cattania. “With numerical models and theory, we try to explain why faults slip the way they do, and the best way to make progress is for modelers and observationalists to talk to each other.”
“What I really like about observational geophysics, and for my science to be useful, is collaborating and interacting with many different people,” says Frank. “Part of that is bringing together the different observational approaches and the constraints that we can generate, and [then] communicating our results to the modelers. More often than not, there's not as much communication as we'd like [between the groups]; so I’m super excited about Camilla being here.”
Share this news article on:
Related links.
- Camilla Cattania
- William Frank
- Department of Earth, Atmospheric and Planetary Science
Related Topics
- Earthquakes
- Computer modeling
- Earth and atmospheric sciences
Related Articles
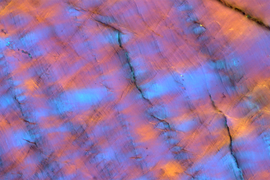
Nanograins make for a seismic shift
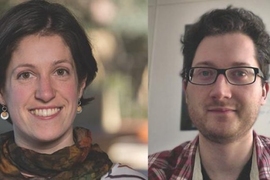
3 Questions: Understanding the Haiti earthquakes
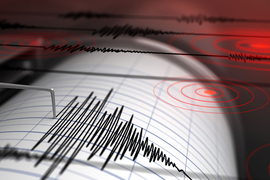
A new approach to preventing human-induced earthquakes
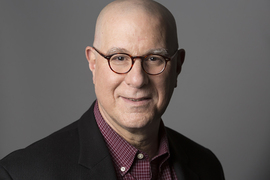
3 Questions: Richard Samuels on Japan’s 3.11 triple disaster and its impact 10 years later
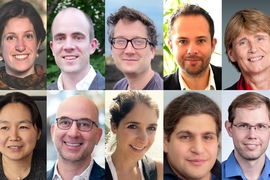
School of Science grows by 10
Previous item Next item
More MIT News
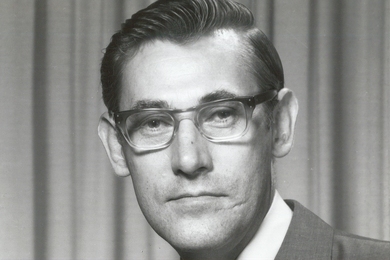
Professor Emeritus David Lanning, nuclear engineer and key contributor to the MIT Reactor, dies at 96
Read full story →
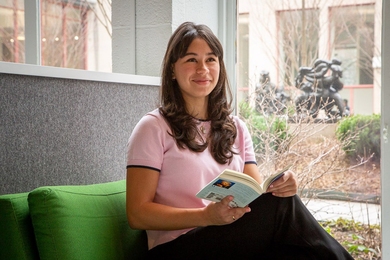
Discovering community and cultural connections
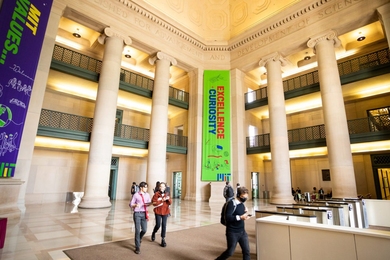
MIT Supply Chain Management Program earns top honors in three 2024 rankings
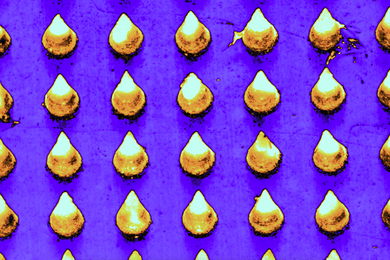
New treatment could reverse hair loss caused by an autoimmune skin disease
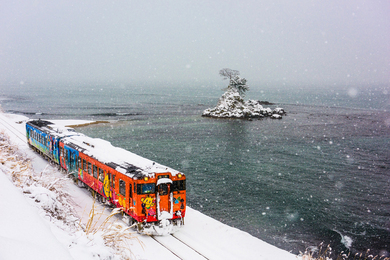
Study: Heavy snowfall and rain may contribute to some earthquakes
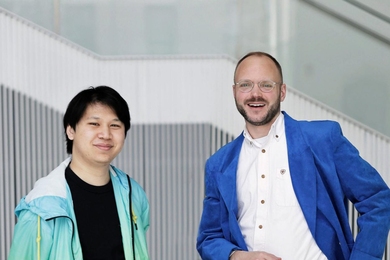
How AI might shape LGBTQIA+ advocacy
- More news on MIT News homepage →
Massachusetts Institute of Technology 77 Massachusetts Avenue, Cambridge, MA, USA
- Map (opens in new window)
- Events (opens in new window)
- People (opens in new window)
- Careers (opens in new window)
- Accessibility
- Social Media Hub
- MIT on Facebook
- MIT on YouTube
- MIT on Instagram
PEER Reports
Two-Dimensional Debris-Fluid-Structure Interaction with the Particle Finite Element Method, PEER Report 2024-04
In addition to tsunami wave loading, tsunami-driven debris can cause significant damage to coastal infrastructure and critical bridge lifelines. Using numerical simulations to predict loads imparted by debris on structures is necessary to supplement the limited number of physical experiments of in-water debris loading. To supplement SPH-FEM (Smoothed Particle Hydrodynamics-Finite Element Method) simulations described in a companion PEER report, fluid-structure-debris simulations using the Particle Finite Element Method (PFEM) show the debris modeling capabilities in OpenSees. A new contact...
- Read more about Two-Dimensional Debris-Fluid-Structure Interaction with the Particle Finite Element Method, PEER Report 2024-04
An International Workshop on Large-Scale Shake Table Testing for the Assessment of Soil-Foundation-Structure System Response for Seismic Safety of DOE Nuclear Facilities, A Virtual Workshop – 17-18 May 2021, PEER Report 2024-03
Aging infrastructure within the US Department of Energy (DOE) and the National Nuclear Security Administration (NNSA) nuclear facilities poses a major challenge to their resiliency against natural phenomenon hazards. Examples of mission-critical facilities located in regions of high seismicity can be found at a number of NNSA sites including Lawrence Livermore National Laboratory, Los Alamos National Laboratory, and the Nevada National Security Site. Most of the nation’s currently operating nuclear facilities have already reached their operating lifetime, and most currently operating...
- Read more about An International Workshop on Large-Scale Shake Table Testing for the Assessment of Soil-Foundation-Structure System Response for Seismic Safety of DOE Nuclear Facilities, A Virtual Workshop – 17-18 May 2021, PEER Report 2024-03
Seismic Performance of Isolated Bridges Under Beyond Design Basis Shaking, PEER Report 2024-02
Seismically isolated highway bridges are expected to provide limited service under a safety evaluation-level ground shaking with minimal to moderate damage. The behavior under shaking beyond design considerations, corresponding to a large return period seismic hazard, is not well understood and could induce significant damage. In these rare events, the seismic isolation system can be subjected to displacement demands beyond its design capacity, resulting in failure of the bearings, exceeding the clearance and pounding against the abutment backwalls, or damage propagating to other primary...
- Read more about Seismic Performance of Isolated Bridges Under Beyond Design Basis Shaking, PEER Report 2024-02
Expert Panel Recommendations for Ergodic Site Amplification in Central and Eastern North America, PEER Report 2017-04
The U.S. Geological Survey (USGS) national seismic hazard maps have historically been produced for a reference site condition of V S30 = 760 m/sec (where V S30 is time averaged shear wave velocity in the upper 30 m of the site). The resulting ground motions are modified for five site classes (A-E) using site amplification factors for peak acceleration and ranges of short- and long-oscillator periods. As a result of Project 17 recommendations, this practice is being revised: (1) maps will be produced for a range of site conditions (as represented by V ...
- Read more about Expert Panel Recommendations for Ergodic Site Amplification in Central and Eastern North America, PEER Report 2017-04
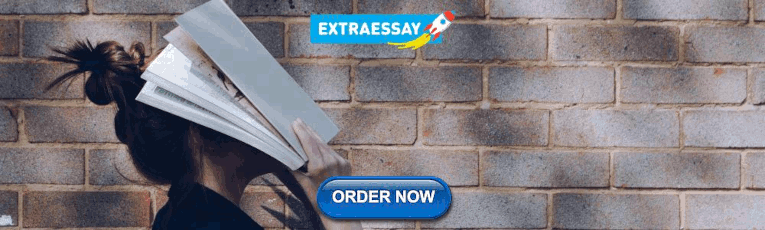
Response Modification of Structures with Supplemental Rotational Inertia, PEER Report 2024-01
Tall, multistory, buildings are becoming increasingly popular in large cities as a result of growing urbanization trends (United Nations Department of Economic and Social Affairs 2018). As cities continue to grow, many of them along the coasts of continents which are prone to natural hazards, the performance of tall, flexible buildings when subjected to natural hazards is a pressing issue with engineering relevance. The performance of structures when subjected to dynamic loads can be enhanced with various response modification strategies which have been traditionally...
- Read more about Response Modification of Structures with Supplemental Rotational Inertia, PEER Report 2024-01
NGA-East Ground-Motion Models for the U.S. Geological Survey National Seismic Hazard Maps, PEER Report 2017-03
The purpose of this report is to provide a set of ground motion models (GMMs) to be considered by the U.S. Geological Survey (USGS) for their National Seismic Hazard Maps (NSHMs) for the Central and Eastern U.S. (CEUS). These interim GMMs are adjusted and modified from a set of preliminary models developed as part of the Next Generation Attenuation for Central and Eastern North-America (CENA) project (NGA-East). The NGA-East objective was to develop a new ground-motion characterization (GMC) model for the CENA region. The GMC model consists of a set of GMMs for median and standard...
- Read more about NGA-East Ground-Motion Models for the U.S. Geological Survey National Seismic Hazard Maps, PEER Report 2017-03
U.S.—New Zealand— Japan International Workshop, Liquefaction-Induced Ground Movement Effects, University of California, Berkeley, California, 2-4 November 2016, PEER Report 2017-02
There is much to learn from the recent New Zealand and Japan earthquakes. These earthquakes produced differing levels of liquefaction-induced ground movements that damaged buildings, bridges, and buried utilities. Along with the often spectacular observations of infrastructure damage, there were many cases where well-built facilities located in areas of liquefaction-induced ground failure were not damaged. Researchers are working on characterizing and learning from these observations of both poor and good performance.
The “Liquefaction-Induced Ground Movements Effects” workshop...
- Read more about U.S.—New Zealand— Japan International Workshop, Liquefaction-Induced Ground Movement Effects, University of California, Berkeley, California, 2-4 November 2016, PEER Report 2017-02
PEER Annual Report 2016, PEER Report 2017-01
The Pacific Earthquake Engineering Research Center (PEER) is a multi-institutional research and education center with headquarters at the University of California, Berkeley. PEER’s mission is to develop, validate, and disseminate performance-based seismic design technologies for buildings and infrastructure to meet the diverse economic and safety needs of owners and society.
The year 2016 began with a change of leadership at PEER. On January 1, Professor Khalid Mosalam became the new PEER Director as Professor Stephen Mahin completed his 6- year term. Also in early 2016, Dr. Yousef...
- Read more about PEER Annual Report 2016, PEER Report 2017-01
Central and Eastern North America Ground-Motion Characterization - NGA-East Final Report, PEER Report 2018-08
This document is the final project report of the Next Generation Attenuation for Central and Eastern North America (CENA) project (NGA-East). The NGA-East objective was to develop a new ground-motion characterization (GMC) model for the CENA region. The GMC model consists of a set of new ground-motion models (GMMs) for median and standard deviation of ground motions and their associated weights to be used with logic-trees in probabilistic seismic hazard analyses (PSHA). NGA-East is a large multidisciplinary project coordinated by the Pacific Earthquake Engineering Research Center (PEER),...
- Read more about Central and Eastern North America Ground-Motion Characterization - NGA-East Final Report, PEER Report 2018-08
An Empirical Model for Fourier Amplitude Spectra using the NGA-West2 Database, PEER Report 2018-07
An empirical ground-motion model (GMM) for shallow crustal earthquakes in California and Nevada based on the NGA-West2 database [Ancheta et al. 2014] is presented. Rather than the traditional response spectrum GMM, this model is developed for the smoothed effective amplitude spectrum (EAS) as defined by PEER [Goulet et al. 2018]. The EAS is the orientation- independent horizontal component Fourier amplitude spectrum (FAS) of ground acceleration. The model is developed using a database dominated by California earthquakes, but takes advantage of crustal earthquake data worldwide to constrain...
- Read more about An Empirical Model for Fourier Amplitude Spectra using the NGA-West2 Database, PEER Report 2018-07
- 1 of 48 View: Taxonomy term (Current page)
- 2 of 48 View: Taxonomy term
- 3 of 48 View: Taxonomy term
- 4 of 48 View: Taxonomy term
- 5 of 48 View: Taxonomy term
- 6 of 48 View: Taxonomy term
- 7 of 48 View: Taxonomy term
- 8 of 48 View: Taxonomy term
- 9 of 48 View: Taxonomy term
- next › View: Taxonomy term
- last » View: Taxonomy term
- Search Menu
- Volume 238, Issue 1, July 2024 (In Progress)
- Volume 237, Issue 3, June 2024
- Advance Access
- Applied and Marine Geophysics
- General Geophysical Methods
- Geodynamics and Tectonics
- Geomagnetism and Electromagnetism
- Gravity, Geodesy and Tides
- Heat Flow and Volcanology
- Rock and Mineral Physics, Rheology
- 100 Influential Papers
- Advance Articles
- Express Letters
- Hunga Volcano Special Issue
- East Anatolia Fault Special Issue
- Special Issues
- Why Publish
- Author Guidelines
- Submission Site
- Read & Publish
- Developing Countries Initiative
- Author Resources
- Self-Archiving policy
- Rights and Permissions
- About Geophysical Journal International
- Editorial Board
- About the Royal Astronomical Society
- About the DGG
- Journals on Oxford Academic
- Books on Oxford Academic

Article Contents
- < Previous
Earthquake prediction: a critical review
- Article contents
- Figures & tables
- Supplementary Data
Robert J. Geller, Earthquake prediction: a critical review, Geophysical Journal International , Volume 131, Issue 3, December 1997, Pages 425–450, https://doi.org/10.1111/j.1365-246X.1997.tb06588.x
- Permissions Icon Permissions
Earthquake prediction research has been conducted for over 100 years with no obvious successes. Claims of breakthroughs have failed to withstand scrutiny. Extensive searches have failed to find reliable precursors. Theoretical work suggests that faulting is a non-linear process which is highly sensitive to unmeasurably fine details of the state of the Earth in a large volume, not just in the immediate vicinity of the hypocentre. Any small earthquake thus has some probability of cascading into a large event. Reliable issuing of alarms of imminent large earthquakes appears to be effectively impossible.
Abelson P.H.
Abercrombie R.E. 1996 . The magnitude-frequency distribution of earthquakes recorded with deep seismometers at Cajon Pass, southern California , Tectonophysics , 261 , 1 – 7 .
Google Scholar
Abercrombie R.E. Brune J.N. 1994 . Evidence for a constant b -value above magnitude 0 in the southern San Andreas, San Jacinto and San Miguel fault zones, and at the Long Valley caldera. California , Geophys. Res. Lett. , 21 , 1647 – 1650 .
Abercrombie R.E. Mori J. 1996 . Occurrence patterns of foreshocks to large earthquakes in the western United States , Nature , 381 , 303 – 307 .
Aceves R.L. Park S.K. 1997 . Cannot earthquakes be predicted ?, Science , 278 , 488 .
Adams R.D. 1976 . The Haicheng, China, earthquake of 4 February 1975: The first successfully predicted major earthquake , Earthq. Eng. Struct. Dyn. , 4 , 423 – 437 .
Adams R.D. 1977 . Earthquake prediction , Nature , 269 , 14 .
Aggarwal Y.P. Sykes L.R. Armbruster J. Sbar M.L. 1973 . Premonitory changes in seismic velocities and prediction of earthquakes . Nature , 241 , 101 – 104 .
Aggarwal Y.P. Sykes L.R. Simpson D.W. Richards P.G. 1975 . Spatial and temporal variations in t s / t p and in P wave residuals at Blue Mountain Lake, New York: Application to earthquake prediction , J. geophys. Res. , 80 , 718 – 732 .
Agnew D.C. Ellsworth W.L. 1991 . Earthquake prediction and long-term hazard assessment , Rei. Geophys. Suppl. , 29 , 877 – 889 .
Agnew D.C. Jones L.M. 1991 . Prediction probabilities from foreshocks , J. geophys. Res. , 96 , 11 959 – 11 971 .
Aki K. 1978 . Progress in Japanese earthquake prediction research , Earthq. Info. Bull. , 10 , 200 – 203 .
Aki K. 1980 . Possibilities of seismology in the 1980's . Bull. seism. Soc. Am. , 70 , 1969 – 1976 .
Aki K. 1989 . Ideal probabilistic earthquake prediction . Tectonophysics , 169 , 197 – 198 .
Aki K. 1995 . Earthquake prediction, societal implications , Rev. Geophys. Suppl. , 33 , 243 – 247 .
Allen C.R. 1976 . Responsibilities in earthquake prediction , Bull. seism. Soc. Am. , 66 , 2069 – 2074 .
Allen C.R. 1982 . Earthquake prediction—1982 overview , Bull. seism. Soc. Am. , 72 , S331 – S335 .
Allen C.R. Helmberger D.V. 1973 . Search for temporal changes in seismic velocities using large explosions in southern California , in Proc. Conf. Tectonic Problems of the San Andreas Fault System . eds Kovach R.L. Nur A. , Vol. 13 , pp. 436 – 445 , Stanford Univ. Publ. Geol. Sci. , Stanford, CA.
Google Preview
Allen C.R. et al. 1968 . The Borrego Mountain, California, earthquake of 9 April 1968: A preliminary report , Bull. seism. Soc. Am. , 58 , 1183 – 1186 .
Allen C.R. (Chairman) Edwards W. Hall W.J. Knopoff L. Raleigh C.B. Savit C.H. Toksöz M.N. Turner R.H. 1976 . Predicting Earthquakes: A Scientific and Technical Evaluation—With Implications for Society . Panel on Earthquake Prediction of the Committee on Seismology, Assembly of Mathematical and Physical Sciences, National Research Council, U.S. National Academy of Sciences , Washington, DC.
Alsop L.E. Oliver J.E. (eds) 1969 . Premonitory phenomena associated with several recent earthquakes and related problems . Eos. Trans. Am. geophys. Un. , 50 , 376 – 410 .
Anderson D.L. 1974 . The Jupiter effect (book review) , Am. Scientist , 62 , 721 – 722 .
Anderson D.L. Whitcomb J.H. 1975 . Time-dependent seismology , J. geophys. Res. , 80 , 1497 – 1503 .
Anderson P.W. 1990 . On the nature of physical laws , Physics Today , 43 ( 12 ), 9 .
Anderson P.W. 1992 . The Reverend Thomas Bayes, needles in haystacks, and the fifth force , Physics Today , 45 ( 1 ), 9 – 11 .
Ando M. 1975 . Possibility of a major earthquake in the Tokai district, Japan, and its pre-estimated seismolectonic effects , Tectonophysics , 25 , 69 – 85 .
Anonymous 1912 . Nature , 90 , 340 .
Anonymous 1922 . Geological Society , Nature , 109 , 361 .
Anonymous 1927 . Earthquake prediction in California , Nature , 119 , 684 .
Anonymous 1931 . Luminous phenomena accompanying earthquakes , Nature , 128 , 155 .
Anonymous 1931 . Active earthquake-faults in Tokyo , Nature , 127 , 796 .
Anonymous 1932 . Luminous phenomena accompanying earthquakes . Nature , 129 , 27 .
Anonymous 1932 . Luminous phenomena accompanying earthquakes , Nature , 130 , 969 .
Anonymous 1933 . Sensitivity of fish to earthquakes , Nature , 132 , 817 .
Anonymous 1933 . Birds and earthquakes , Nature , 132 , 964 .
Anonymous 1972 . Predicting earthquakes , Nature , 237 , 305 .
Anonymous 1972 . Scientific activities of IASPEI during the fifteenth general assembly , Eos. Trans. Am. geophys. Un. , 53 , 235 – 236 .
Anonymous 1973 . Precursory V p / V s changes , Nature , 243 , 380 .
Anonymous 1973 . Using prediction properly , Nature , 245 , 174 .
Anonymous 1973 . No dilatancy , Nature , 246 , 332 – 333 .
Anonymous 1974 . New method of predicting earthquakes , Nature , 247 , 253 .
Anonymous 1975 . Progress of U.S.-USSR cooperative program in earthquake prediction , Eos. Trans. Am. geophys. Un. , 56 , 524 .
Anonymous 1975 . The Chinese dimension , Nature , 258 , 277 .
Anonymous 1976 . Still a lot to learn from the Chinese , Nature , 262 , 431 .
Anonymous 1978 . Amateur earthquake predictions , Eos. Trans. Am. geophys. Un. , 59 , 963 .
Anonymous 1982 . Cooperative seismology studies between the U.S. and the People's Republic of China , Earthq. Info. Bull. , 14 , 144 – 146 .
Anonymous 1995 . Japan's seismic tragedy at Kobe , Nature , 373 , 269 .
Anonymous 1996 . Money doesn't buy quake prediction faith , Science , 271 , 915 .
Aoki H. 1996 . Critical state of the crust and earthquake precursors . Kagaku , 65 , 232 – 240 (in Japanese).
Asada T. (ed.) 1982 . Earthquake Prediction Techniques—Their Application in Japan , University of Tokyo Press , Tokyo.
Ashida M. 1997 . The large-scale earthquake countermeasures act should be repealed immediately , Ronza , Feb. 1997, 50 – 55 (in Japanese).
Aster R.C. Shearer P.M. Berger J. 1990 . Quantitative measurements of shear wave polarizations at the Anza seismic network, southern California: Implications for shear wave splitting and earthquake prediction , J. geophys. Res. , 95 , 12 449 – 12 473 .
Aster R.C. Shearer P.M. Berger J. 1991 . Reply , J. geophys. Res. , 96 , 6415 – 6419 .
Aziz A. 1942 . Luminous phenomena accompanying the Cyprus earthquake, January 20, 1941 , Nature , 149 , 640 .
Bak P. 1996 . How Nature Works: the Science of Self-Organized Criticality, Copernicus . New York, NY.
Bak P. Tang C. 1989 , Earthquakes as a self-organized critical phenomenon , J. geophys. Res. , 94 , 15 635 – 15 637 .
Bak P. Tang C. Wiesenfeld K. 1988 . Self-organized criticality , Phys. Rev. , A 38 , 364 – 374 .
Bakun W.H. 1987 . Future earthquakes , Rev. Geophys. , 25 , 1135 – 1138 .
Bakun W.H. Lindh A.G. 1985 . The Parkfield, California, earthquake prediction experiment , Science , 229 , 619 – 624 .
Bakun W.H. Lindh A.G. 1985 . The Parkfield, California, prediction experiment , Earthq. Predict. Res. , 3 , 285 – 304 .
Bakun W.H. McEvilly T.V. 1979 . Earthquakes near Parkfield, California: Comparing the 1934 and 1966 sequences , Science , 205 , 1375 – 1377 .
Bakun W.H. McEvilly T.V. 1984 . Recurrence models and Parkfield, California, earthquakes , J. geophys. Res. , 89 , 3051 – 3058 .
Ben-Menahem A. 1995 . A concise history of mainstream seismology – origins, legacy, and perspectives , Bull. seism. Soc. Am. , 85 , 1202 – 1225 .
Ben-Zion Y. Rice J.R. 1995 . Slip patterns and earthquake populations along different classes of faults in elastic solids , J. geophys. Res. , 100 , 12 959 – 12 983 .
Berckhemer H. (ed.) 1985 . Special issue: Proceedings of the ESC symposium on earthquake prediction research , Earthq. Predict. Res. , 3 , 69 – 201 .
Bernard P. Pinettes P. Hatzidimitriou P.M. Scordilis E.M. Veis G. Paraskevas M. 1997 . From precursors to prediction: A few recent cases from Greece . Geophys. J. Int. , 131 , 467 – 477 (this issue).
Beroza G. 1996 . Rupture history of the earthquake from high-frequency strong-motion data , in The Loma Prieta, California Earthquake of October 17, 1989—Main-shock Characteristics , U.S. Geological Survey Professional Paper 1550-A, pp. A9 – A32 .
Beroza G. 1997 . Earthquake seismology , Geotimes , 42 ( 2 ), 53 – 54 .
Beroza G. Ellsworth W.L. 1996 . Properties of the seismic nucleation phase , 261 , 209 – 227 .
Berry M.J. (ed.) 1989 . Special issue: Earthquake hazard assessment and prediction , Tectonophysics , 167 , 81 – 361 .
Board on Earth Sciences 1986 . A Proposed Initiative for Capitalizing on the Parkfield, California, Earthquake Prediction , Commission on Physical Sciences, Mathematics, and Resources , National Research Council, National Academy Press , Washington, DC.
Bock Y. 1994 . Crustal deformation and earthquakes , Geotimes , 39 ( 6 ), 16 – 18 .
Bock Y. et al. 1993 . Detection of crustal deformation from the Landers earthquake sequence using continuous geodetic measurements , Nature , 361 , 337 – 340 .
Bolt B.A. 1974 . Earthquake studies in the People's Republic of China , Eos. Trans. Am. Geophys. Un. , 55 , 108 – 117 .
Bolt B.A. 1975 . Book review of The Jupiter Effect , Physics Today , 28 ( 4 ), 74 – 75 .
Boulanger Y.D. Vyskočil P. (eds), 1992 . Special issue on Geodesy—Seismology: Deformations and Prognosis , Tectonophysics , 202 , 97 – 290 .
Bowman J.R. 1997 . Case 22: A seismicity precursor to a sequence of M , 6.3–6.7 midplate earthquakes in Australia , Pure appl. Geophys. , 149 , 61 – 78 .
Brace W.F. 1975 . The physical basis for earthquake prediction , Technology Review , March/April 1975 issue , 26 – 29 .
Brady B.T. 1976 . Theory of earthquakes – IV. General implications for earthquake prediction , Pure appl. Geophys. , 114 , 1031 – 1082 .
Brune J.N. 1974 . Current status of understanding quasi-permanent fields associated with earthquakes , Eos. Trans. Am. geophys. Un. , 55 , 820 – 827 .
Brune J.N. 1979 . Implications of earthquake triggering and rupture propagation for earthquake prediction based on premonitory phenomena . J. geophys. Res. , 84 , 2195 – 2198 .
Burts E. et al. 1983 . Earthquake prediction and preparedness in Japan , Earthq. Info. Bull. , 15 , 142 – 147 .
Buskirk R.E. Frohlich C. Latham G.V. 1981 . Unusual animal behaviour before earthquakes: A review of possible sensory mechanisms . Rev. Geophys. Space Phys. , 19 , 247 – 270 .
Carlowicz M. 1996 . Japan, U.S. plan joint earthquake research , Eos. Trans. Am. geophys. Un. , 77 , 377 – 378 .
Carter L.J. 1966 . Earthquake prediction: ESSA and USGS vie for leadership , Science , 151 , 181 – 183 .
Castle R.O. 1978 . Leveling surveys and the southern California uplift , Earthq. Info. Bull. , 10 , 88 – 92 .
Castle R.O. Gilmore T.D. 1992 . A revised configuration of the southern California uplift , Geol. Soc. Am. Bull. , 104 , 1577 – 1591 .
Castle R.O. Alt J.N. Savage J.C. Balzars E.I. 1974 . Elevation changes preceding the San Fernando earthquake of February 9, 1971 , Geology , 2 , 61 – 66 .
Castle R.O. Church J.P. Elliott M.R. Morrison N.L. 1975 . Vertical crustal movements preceding and accompanying the San Fernando earthquake of February 9, 1971: A summary , Tectonophysics , 29 , 127 – 140 .
Castle R.O. Church J.P. Elliott M.R. 1976 . Aseismic uplift in southern California , Science , 192 , 251 – 253 .
Castle R.O. Elliott M.R. Gilmore T.D. Mark R.K. Newman E.B. Tinsley J.C. III 1981 . Aseismic uplift in California , Science , 213 , 246 – 247 .
Castle R.O. Church J.P. Elliot M.R. Gilmore T.D. Mark R.K. Newman E.B. Tinsley J.C. III 1983 . Comment on ‘The impact of refraction correction on leveling interpretations in southern California,’ by William E. Strange , J. geophys. Res. , 88 , 2508 – 2512 .
Castle R.O. Brown B.W. Jr. Gilmore T.D. Mark R.K. Wilson R.C. 1983 . An examination of the southern California field test for the systematic accumulation of the optical refraction error in geodetic leveling , Geophys. Res. Lett. , 10 , 1081 – 1084 .
Castle R.O. Elliott M.R. Church J.P. Wood S.H. 1984 . The Evolution of the Southern California Uplift, 1955 Through 1976 , U.S. Geological Survey Professional Paper 1342, Washington, DC.
Castle R.O. Elliott M.R. Gilmore T.D. 1987 . An Early-20th Century Uplift in Southern California , U.S. Geological Survey Professional Paper 1362, Washington, DC.
Chan W. 1989 . Congressional hearing looks at lessons of quake , AGU Daily in San Francisco. 5 December 1989, p. 1 .
Chen Y.-T. Chen Z.-L. Wang B.Q. 1992 . Seismological studies of earthquake prediction in China: a review , in Earthquake Prediction – Proceedings of the International School of Solid Earth Geophysics, 5th course , eds Dragoni M. Boschi E. pp. 71 – 109 , Il Cigno Galileo Galilei , Rome.
Chen Z.-L. 1986 . Earthquake prediction research in China: Status and prospects , J. Phys. Earth , 34 , S1 – S11 .
Chi S.C. Reilinger R.E. Brown L.D. Oliver J.E. 1980 . Leveling circuits and crustal movement , J. geophys. Res. , 85 , 1469 – 1474 .
Chu F.-M. 1976 . An outline of prediction and forecast of Haicheng earthquake of M=7.3 . in Proc. Lectures by the Seismological Delegation of the People's Republic of China , ed. Muller P.M. Jet Propulsion Laboratory , Pasadena. CA, Rept SP 43–32, Aug. 1, 1976, pp. 11 – 19 .
Chu J.J. Gui X. Dai J. Marone C. Spiegelman M.W. Seeber L. Armbruster J.G. 1996 . Geoelectrical signals in China and the earthquake generation process , J. geophys. Res. , 101 , 13 869 – 13 882 .
Coe R.S. 1971 . Earthquake prediction program: the People's Republic of China , Eos. Trans. Am. geophys. Un. , 52 , 940 – 943 .
Crampin S. 1987 . The basis for earthquake prediction . Geophys. J. R. astr. Soc. , 91 , 331 – 347 .
Crampin S. 1988 . Extensive-dilatancy anisotropy: New earthquake prediction tool , Eos. Trans. Am. geophys. Un. , 69 , 729 .
Crampin S. Zatsepin S.V. 1997 . Changes of strain before earthquakes: The possibility of routine monitoring of both long-term and short-term precursors . J. Phys. Earth , 45 , 41 – 66 .
Crampin S. Booth D.C. Evans R. Peacock S. Fletcher J.B. 1991 . Comment on ‘Quantitative measurements of shear wave polarizations at the Anza seismic network, southern California: Implications for shear wave splitting and earthquake prediction,’ by Richard C. Aster, Peter M. Shearer, and Jon Berger . J. geophys. Res. , 96 , 6403 – 6414 .
Craymer M.R. Vaniíček P. 1986 . Further analysis of the 1981 southern California field test for leveling refraction , J. geophys. Res. , 91 , 9045 – 9055 .
Craymer M.R. Vaniíček P. 1989 . Comment on ‘Saugus-Palmdale, California, field test for refraction error in historical leveling surveys’ by R.S. Stein, C.T. Whalen, S.R. Holdahl. W.E. Strange, and W. Thatcher, and Reply to Comment on ‘Further analysis of the 1981 southern California field test for levelling refraction by M.R. Craymer and P. Vaniíček’ by R.S. Stein, C.T. Whalen, S.R. Holdahl, W.E. Strange, and W. Thatcher , J. geophys. Res. , 94 , 7667 – 7672 .
Dalrymple G.B. 1991 . Good press for bad science , Eos. Trans. Am. geophys. Un. , 72 , 41 .
Dambara T. 1981 . Geodesy and earthquake prediction , in Current Research in Earthquake Prediction I , pp. 167 – 220 , ed. Rikitake T. D. Reidel , Dordrecht/CAPJ, Tokyo.
Davies D. 1973 . Predicting earthquakes , Nature , 245 , 121 – 122 .
Davies D. 1974 . Quake prediction no bonanza , Nature , 249 , 7 .
Davies D. 1975 . Earthquake prediction in China , Nature , 258 , 286 – 287 .
Davis J.F. Somerville P. 1982 . Comparison of earthquake prediction approaches in the Tokai area of Japan and in California , Bull. seism. Soc. Am. , 72 , S367 – S392 .
Davis P.M. Jackson D.D. Kagan Y.Y. 1989 . The longer it has been since the last earthquake, the longer the expected time until the next ?, Bull. seism. Soc. Am. , 79 , 1439 – 1456 .
DeMets C. 1997 . Afterslip no longer an afterthought , Nature , 386 , 549 – 550 .
de Montalk R.W. 1934 . Earthquakes: The futility of predicting them , Bull. seism. Soc. Am. , 24 , 100 – 108 .
Deng Q. Jiang P. Jones L.M. Molnar P. 1981 . A preliminary analysis of reported changes in ground water and anomalous animal behaviour before the 4 February 1975 Haicheng earthquake , in Earthquake Prediction: An International Review Simpson D.W. Richards P.G. , eds Ewing Monograph Series 4, pp. 543 – 565 . Am. Geophys. Un. , Washington, DC.
Diaconis P. 1978 . Statistical problems in ESP research , Science , 201 , 131 – 136 .
Dieterich J.H. 1978 . Preseismic fault slip and earthquake prediction . J. geophys. Res. , 83 , 3940 – 3948 .
Dieterich J.H. Brace W.F. 1975 . Earthquake-related experimental studies in the USSR . Eos. Trans. Am. geophys. Un. , 56 , 221 – 223 .
Dixon T.H. Mao A. Stein S. 1996 . How rigid is the stable interior of the North American plate ?, Geophys. Res. Lett. , 23 , 3035 – 3038 .
Dodge D.A. Beroza G.C. Ellsworth W.L. 1996 . Detailed observations of California foreshock sequences: Implications for the earthquake initiation process , J. geophys. Res. , 101 , 22 371 – 22 392 .
Dologlou E. 1993 . A three year continuous sample of officially documented predictions issued in Greece using the VAN method: 1987–1989 , Tectonophysics , 224 , 189 – 202 .
Dragoni M. Boschi E. (eds), 1992 . Earthquake Prediction - Proceedings of the International School of Solid Earth Geophysics, 5th course , Il Cigno Galileo Galilei , Rome.
Drakopoulos J. Stavrakakis G.N. 1996 . A false alarm based on electrical activity recorded at a VAN-station in northern Greece in December 1990 . Geophys. Res. Lett. , 23 , 1355 – 1358 .
Dziewonski A.M. Ekström G. Salganik M.P. 1996 . Centroid-moment tensor solutions for July-September 1995 , Phys. Earth planet. Inter. , 97 , 3 – 13 .
Echevarria J.A. Norton K.A. Norton R.D. 1986 . The socio-economic consequences of earthquake prediction: A case study in Peru , Earthq. Pred. Res. , 4 , 175 – 195 .
Ellsworth W.L. 1993 . Getting beyond numerology , Nature , 363 , 206 – 207 .
Ellsworth W.L. Beroza G.C. 1995 . Seismic evidence for a nucleation phase , Science , 268 , 851 – 855 .
Ellsworth W.L. et al. 1973 . Point Mugu, California, earthquake of 21 February 1973 and its aftershocks , Science , 182 , 1127 – 1129 .
Evans J.W. 1923 . Earthquake warnings , Nature , 112 , 538 .
Evans J.W. 1927 . Earthquake warnings , Nature , 120 , 619 .
Evernden J.F. 1982 . Earthquake prediction: What we have learned, and what we should do now , Bull. seism. Soc. Am. , 72 , S343 – S349 .
Evison F.F. (Rapporteur) 1984 . Code of practice for earthquake prediction , IUGG Chronicle. No. 165 , 26 – 29 (Feb.).
Evison F. (ed.) 1989 . Special section on earthquake hazard and prediction: Time-variable earthquake hazard , Tectonophysics , 169 , 154 – 213 .
Evison F.F. Rhoades D.A. 1993 . The precursory earthquake swarm in New Zealand: hypothesis tests , New Zealand J. Geol. Geophys. , 36 , 51 – 60 (correction p. 267).
Finn R. 1992 . Rumblings grow about Parkfield in wake of first earthquake prediction , Nature , 359 , 761 .
Franklin A. 1993 . The Rise and Fall of the Fifth Force , Am. Inst. Phys. , New York, NY.
Franks F. 1981 . Polywater , MIT Press , Cambridge, MA.
Fraser-Smith A.C. Bernardi A. McGill P.R. Ladd M.E. Heliwell R.A. Villard O.G. Jr. 1990 . Low-frequency magnetic field measurements near the epicentre of the M, 7.1 Loma Prieta earthquake , Geophys. Res. Lett. , 17 , 1465 – 1468 .
Fraser-Smith A.C. Bernardi A. Heliwell R.A. McGill P.R. Villard O.G. Jr. 1993 . Analysis of low-frequency-electromagnetic-field measurements near the epicentre , in The Loma Prieta, California, Earthquake of October 17, 1989—Preseismic Observations , pp. C17 – C25 , ed. Johnston M. J. S. U.S. Geological Survey Professional Paper 1550-C. Washington, DC.
Gao W.-X. Gao Q.-H. 1995 . Intensifying earthquake prediction in the light of action of international decade for natural disaster reduction . Earthq. Res. China , 9 , 211 – 217 .
Garwin L. 1989 . A successful prediction ?, Nature , 341 , 677 .
Garza T. Lomnitz C. 1979 . The Oaxaca gap: A case history , Pure appl. Geophys. , 117 , 1187 – 1194 .
Geller R.J. 1991 . Shake-up for earthquake prediction , Nature , 352 , 275 – 276 .
Geller R.J. 1991 . Unpredictable earthquakes , Nature , 353 , 612 .
Geller R.J. (ed.) 1996 . Special issue: ‘Debate on “VAN ”’, Geophys. Res. Lett. , 23 , 1291 – 1452 .
Geller R.J. 1996 . VAN: A critical evaluation , in A Critical Review of VAN . pp. 155 – 238 , ed. Lighthill J. World Scientific , Singapore.
Geller R.J. 1996 . One year after the Kobe earthquake , Shincho 45 . Feb. 1996 issue , 22 – 31 (in Japanese).
Geller R.J. 1997 . Earthquakes: Thinking about the unpredictable , Eos. Trans. Am. geophys. Un. , 78 , 63 – 67 .
Geller R.J. 1997 . Predictable publicity , Astronomy & Geophysics. J. R. Astr. Soc. Feb.March 1997 issue, 38 ( 1 ), 16 – 18 (reprinted 1997, Seism. Res. Lett., 68 , 477 – 480 ).
Geller R.J. Jackson D.D. Kagan Y.Y. Mulargia F. 1997 . Earthquakes cannot be predicted , Science , 275 , 1616 – 1617 .
Geller R.J. Jackson D.D. Kagan Y.Y. Mulargia F. 1997 . Cannot earthquakes be predicted ?, Science , 278 , 488 – 490 .
Geographical Survey Institute 1979 . Summary of the First 10 Years of the Coordinating Committee for Earthquake Prediction , Tsukuba (in Japanese).
Geschwind C.-H. 1997 . 1920s prediction reveals some pitfalls of earthquake forecasting , Eos. Trans. Am. geophys. Un. , 78 , 401 – 412 .
Giesecke A.A. 1983 . Case history of the Peru prediction for 1980–81 , in Proc. Seminar Earthquake Prediction Case Histories (Geneva, 12–15 October 1982) , pp. 51 – 75 , convened by Office of U.N. Disaster Relief Co-ordinator and UNESCO , UNDRO/83/33, GE.83–03321.
Gilbert G.K. 1909 . Earthquake forecasts , Science , 29 , 121 – 138 .
Gladwin M.T. Gwyther R.L. Higbie J.W. Hart R.G. 1991 . A medium term precursor to the Loma Prieta earthquake ?, Geophys. Res. Lett. , 18 , 1377 – 1380 .
Gokhberg M.B. Morgounov V.A. Yoshino T. Tomizawa I. 1982 . Experimental measurement of electromagnetic emissions possibly related to earthquakes in Japan , J. geophys. Res. , 87 , 7824 – 7828 .
Gokhberg M.B. Gufeld I.L. Rozhnoy A.A. Marenko V.F. Yampolsky V.S. Ponomarev E.A. 1989 . Study of seismic influence on the ionosphere by super long-wave probing of the Earth-ionosphere waveguide , Phys. Earth planet. Inter. , 57 , 64 – 67 .
Gokhberg M.B. Morgounov V.A. Pokhotelov O.A. 1995 . Earthquake Prediction: Seismoelectromagnetic Phenomena , Gordon & Breach , Amsterdam.
Goodstein D.L. 1993 . Richard P. Feynman, teacher , in ‘Most of the Good Stuff,’ Memories of Richard Feynman , pp. 115 – 124 , eds Brown L.M. Rigden J.S. Am. Inst. Phys. , New York, NY.
Gori P.L. 1993 . The social dynamics of a false earthquake prediction and the response by the public sector , Bull. seism. Soc. Am. , 83 , 963 – 980 .
Grant L.B. 1996 . Uncharacteristic earthquakes on the San Andreas Fault , Science , 272 , 826 – 827 .
Greathouse L. 1980 . Palmdale bulge still a puzzle . Eos. Trans. Am. geophys. Un. , 61 , 515 – 516 .
Gribben J. 1971 . Relation of sunspot and earthquake activity , Science , 173 , 558 .
Gribben J. 1975 . Predicting earthquakes , Physics Today , 28 ( 10 ), 13 – 15 .
Gribben J. 1976 . The author comments , Physics Today , 29 ( 6 ), 11 – 13 .
Gribben J.R. Plagemann S.H. 1974 . The Jupiter Effect , MacMillan , London.
Gribben J. Plagemann S. 1975 . Response to Meeus . Icarus , 26 , 268 – 269 .
Griggs D.T. Jackson D.D. Knopoff L. Shreve R.L. 1975 . Earthquake prediction: Modeling the anomalous V p / V s source region , Science , 187 , 537 – 540 .
Gruszow S. Rossignol J.C. Tzanis A. LeMouël J.L. 1996 . Identification and analysis ofelectromagnetic signals in Greece: the case of the Kozani earthquake VAN prediction , Geophys. Res. Lett. , 23 , 2025 – 2028 .
Gu G.-X. 1981 . Strategy of earthquake prediction in China (A personal view) , in Earthquake Prediction: An International Review eds Simpson D.W. Richards P.G. Ewing Monograph Series 4 , pp. 667 – 670 , Am. Geophys. Un. , Washington, DC.
Guha S.K. Patwardhan A.M. (eds), 1988 . Earthquake Prediction—Present Status , Proceedings of a symposium held in Pune, India, July 1986, Registrar, University of Poona, Pune, India.
Guidelines for earthquake predictors 1983 . Bull. seism. Soc. Am. , 73 , 1955 – 1956 .
Gupta H.K. 1997 . Meeting focuses on catastrophic Asian earthquakes , Eos. Trans. Am. geophys. Un. , 78 , 147 . (Correction, p. 166 .).
Gwyther R.L. Gladwin M.T. Mee M. Hart R.H.G. 1996 . Anomalous shear strain at Parkfield during 1993–94 , Geophys. Res. Lett. , 23 , 2425 – 2428 .
Haase J.S. Shearer P.M. Aster R.C. 1995 . Constraints on temporal variations in velocity near Anza, California, from analysis of similar event pairs , Bull. seism. Soc. Am. , 85 , 194 – 206 .
Hager B.H. (chair) Cornell C.A. Medigovich W.M. Mogi K. Smith R.M. Tobin L.T. Stock J. Weldon R. 1994 . Earthquake Research at Parkfield, California, for 1993 and Beyond—Report of the NEPEC [National Earthquake Prediction Evaluation Council] Working Group , U.S. Geological Survey Circular , 1116 .
Hagiwara T. 1997 . Earthquake Prediction and Damage . Maruzen , Tokyo (in Japanese).
Hagiwara T. Rikitake T. 1967 . Japanese program on earthquake prediction , Science , 157 , 761 – 768 .
Hales A.L. Suzuki Z. (eds), 1982 . Special Issue: Earthquake prediction , Tectonophysics , 85 , 1 – 148 .
Hamada K. 1991 . Unpredictable earthquakes , Nature , 353 , 611 – 612 .
Hamada K. 1992 . Present state of earthquake prediction system in Japan , in Earthquake Prediction—Proceedings of the International School of Solid Earth Geophysics, 5th course , pp. 33 – 69 , eds Dragoni M. Boschi E. Il Cigno Galileo Galilei , Rome.
Hamilton R.M. 1974 . Earthquake prediction and public reaction , Eos. Trans. Am. geophys. Un. , 55 , 739 – 742 .
Hammond A.L. 1971 . Earthquake prediction and control , Science , 173 , 316 .
Hammond A.L. 1973 . Earthquake predictions: Breakthrough in theoretical insight ?, Science , 180 , 851 – 853 .
Hammond A.L. 1973 . Earthquake prediction (II): Prototype instrumental networks , Science , 180 , 940 – 941 .
Hammond A.L. 1974 . Dilatancy: Growing acceptance as an earthquake mechanism , Science , 184 , 551 – 552 .
Hammond A.L. 1975 . Earthquake prediction: Progress in California, hesitation in Washington , Science , 187 , 419 – 420 .
Hammond A.L. 1976 . Earthquakes: An evacuation in China, a warning in California , Science , 192 , 538 – 539 .
Hanks T.C. 1985 . The National Earthquake Hazards Reduction Program—Scientific Status , U.S. Geological Bulletin 1659.
Hanks T.C. Kanamori H. (eds), 1979 . Special section: Fault mechanics and its relation to earthquake prediction , J. geophys. Res. , 84 , 2145 – 2370 .
Hanks T.C. Krawinkler H. 1991 . The 1989 Loma Prieta earthquake and its effects: Introduction to the special issue , Bull. seism. Soc. Am. , 81 , 1415 – 1423 .
Hao J.-C. 1996 . The sixth symposium of the Seismological Society of China held at Zhangjiajie, Hunan Province, China , Acta seism. Sinica , 9 , 695 – 696 .
Harris R.A. 1997 . The Loma Prieta, California, Earthquake of October 17, 1989-Forecasts . U.S. Geological Survey Professional Paper , 1550-B , in press.
Harris R.A. Segall P. 1987 . Detection of a locked zone at depth on the Parkfield, California, segment of the San Andreas fault , J. geophys. Res. , 92 , 7945 – 7962 .
Harris R.A. Archuleta R.J. 1988 . Slip budget and potential for a M7 earthquake in Central California , Geophys. Res. Lett. , 15 , 1215 – 1218 .
Hatzfeld D. et al. 1995 . The Kozani-Grevena (Greece) earthquake of May 13, 1995. M s =6.6. Preliminary results of a field multidisciplinary survey , Seism. Res. Lett. , 66 ( 6 ), 61 – 70 .
Hayakawa M. Fujinawa Y. (eds), 1994 . Electromagnetic Phenomena Related to Earthquake Prediction . Terrapub , Tokyo.
Healy J.H. Pakiser L.C. 1971 . Man-made earthquakes and earthquake prediction , Eos. Trans. Am. geophys. Un. ( Suppl. , U.S. Natl. Rept. 1967–1971, 15th IUGG Genl. Assmbl.). 52 , IUGG 171–IUGG 174.
Healy J.H. 1975 . Recent highlights and future trends in research on earthquake prediction and control , Rev. Geophys. Space Phys. , 13 , 361 – 364 ; 390 – 392 .
Heki K. Miyazaki S. Tsuji H. 1997 . Silent fault slip following an interplate thrust earthquake at the Japan trench , Nature , 386 , 595 – 598 .
Hickman S. Sibson R. Bruhn R. (eds), 1995 . Special section: Mechanical involvement of fluids in faulting , J. geophys. Res. , 100 , 12 831 – 13 132 .
Hill D.P. et al. 1993 . Seismicity remotely triggered by the magnitude 7.3 Landers, California, earthquake , Science , 260 , 1617 – 1623 .
Hirose H. 1985 . Earthquake prediction in Japan and the United States , Intl. J. Mass Emergencies Disasters , 3 , 51 – 66 .
Hobbs W.H. 1907 . Earthquakes , Appleton , New York, NY.
Hodgson E.A. 1923 . A proposed research into the possibilities of earthquake prediction , Bull. seism. Soc. Am. , 13 , 100 – 104 .
Holdahl S.R. 1982 . Recomputation of vertical crustal motions near Palmdale, California. 1959–1975 , J. geophys. Res. , 87 , 9374 – 9388 .
Huebsch I.O. 1978 . Comment , Eos. Trans. Am. geophys. Un. , 59 , 2 .
Hughes D.W. 1977 . Planetary alignments don't cause earthquakes , Nature , 265 , 13 .
Huizenga J.R. 1992 . Cold Fusion: The Scientific Fiasco of the Century , University of Rochester Press , Rochester, NY.
Hunter R.N. 1976 . Earthquake prediction: Fact and fallacy , Earthq. Info. Bull. , 8 ( 5 ), 24 – 25 .
Hunter R.N. Derr J.S. 1978 . Prediction monitoring and evaluation program: a progress report , Earthq. Info. Bull. , 10 , 93 – 96 .
Igarashi G. et al. 1995 . Ground-water radon anomaly before the Kobe earthquake in Japan . Science , 269 , 60 – 61 .
Iizuka S. 1976 . Changes in V p /V s before the Tokachi-oki earthquake of May 16, 1968 off NE Japan , Nature , 260 , 415 – 417 .
Imamura A. 1937 . Theoretical and Applied Seismology , Maruzen , Tokyo.
Ip W.H. 1976 . Chinese records on the correlation of heliocentric planetary alignments and earthquakes activities , Icarus , 29 , 435 – 436 .
IPSAD 1997 . Abstracts, United Nations International Conference on Integration of Public Administration and Science of Disasters, 20–28 Jan . 1979, Beijing, U.N. Global Programme for the Integration of Public Administration and Science of Diasters .
Ishibashi K. 1977 . Re-examination of a great earthquake expected in the Tokai district, central Japan—possibility of the ‘Suruga Bay earthquake ’, Rep. Coord. Comm. Earthq. Pred. , 17 , 126 – 132 (in Japanese).
Ishibashi K. 1981 . Specification of a soon-to-occur seismic faulting in the Tokai District. Central Japan, based upon seismotectonics , in Earthquake Prediction: An International Review , eds Simpson D.W. Richards P.G. , Ewing Monograph Series, 4 , 297 – 332 , Am. Geophys. Un. , Washington, DC.
Ishibashi K. 1985 . Possibility of a large earthquake near Odawara, central Japan, preceding the Tokai earthquake , Earthq. Predict. Res. , 3 , 319 – 344 .
Ito K. Matsuzaki M. 1990 . Earthquakes as self-organized critical phenomena , J. geophys. Res. , 95 , 6853 – 6860 .
Jackson D.D. 1996 . Hypothesis testing and earthquake prediction , Proc. Nat. Acad. Sci. USA , 93 , 3772 – 3775 .
Jackson D.D. 1996 . Earthquake prediction evaluation standards applied to the VAN method . Geophys. Res. Lett. , 23 , 1363 – 1366 .
Jackson D.D. Lee W.B. Liu C.-C. 1980 . Aseismic uplift in southern California: An alternative explanation , Science , 210 , 534 – 536 .
Jackson D.D. Lee W.B. Liu C.C. 1981 . Height dependent errors in southern California leveling , in Earthquake Prediction: An International Review , eds Simpson D.W. Richards P.G. , Ewing Monograph Series 4 , pp. 457 – 472 , Am. Geophys. Un. , Washington, DC.
Jackson D.D. Lee W.B. Liu C.-C. 1981 . Aseismic uplift in southern California , Science , 213 , 247 .
Jackson D.D. Cheng A. Liu C.C. 1983 . Tectonic motions and systematic errors in leveling and trilateration data for California , Tectonophysics , 97 , 73 – 83 .
Johnson H.O. Agnew D.C. 1995 . Monument motion and measurement of crustal velocities , Geophys. Res. Lett. , 22 , 2905 – 2908 .
Johnston M.J.S. (ed.) 1993 . The Loma Prieta, California, Earthquake of October 17, 1989—Preseismic Observations , U.S. Geological Survey Professional Paper 1550-C. Washington, DC.
Johnston M.J.S. 1997 . Review of electrical and magnetic fields accompanying seismic and volcanic activity , Surv. Geophys. , 18 , 441 – 475 .
Johnston M.J.S. Parrot M. (eds), 1989 . Special issue: Seismoelectromagnetic effects , Phys. Earth planet. Inter. , 57 , 1 – 177 .
Johnston M.J.S. Parrot M. (eds), 1993 . Special issue: Seismo-electromagnetic effects , Phys. Earth planet. Inter. , 77 , 1 – 141 .
Johnston M.J.S. Linde A.T. Gladwin M.T. Borcherdt R.D. 1987 . Fault failure with moderate earthquakes , Tectonophysics , 144 , 189 – 206 .
Johnston M.J.S. Linde A.T. Gladwin M.T. 1990 . Near-field high resolution strain measurements prior to the October 18, 1989 Loma Prieta M, 7.1 earthquake , Geophys. Res. Lett. , 17 , 1777 – 1780 .
Jones L.M. Hauksson E. 1997 . The seismic cycle in southern California: Precursor or response ?, Geophys. Res. Lett. , 24 , 469 – 472 .
Jones L.M. Molnar P. 1979 . Some characteristics of foreshocks and their possible relationship to earthquake prediction and premonitory slip on faults . J. geophys. Res. , 84 , 3596 – 3608 .
Jones L.M. Wang B.-Q. Xu S.-X. Fitch T.J. 1982 . The foreshock sequence of the February 4, 1975 Haicheng earthquake (M = 7.3) , J. geophys. Res. , 87 , 4575 – 4584 .
Jordan T.H. 1997 . Is the study of earthquakes a basic science ?, Seism. Res. Lett. , 68 , 259 – 261 .
Kagan Y.Y. 1994 . Observational evidence for earthquakes as a nonlinear dynamic process . Physica D , 77 , 160 – 192 .
Kagan Y.Y. 1996 . VAN earthquake predictions—an attempt at statistical evaluation . Geophys. Res. Lett. , 23 , 1315 – 1318 .
Kagan Y.Y. 1996 . Comment on ‘The Gutenberg-Richter or characteristic earthquake distribution, which is it?’ by S. G. Wesnousky , Bull. seism. Soc. Am. , 86 , 274 – 285 .
Kagan Y.Y. 1997 . Are earthquakes predictable ?, Geophys. J. Int. , 131 , 505 – 525 (this issue).
Kagan Y.Y. 1997 . Statistical aspects of Parkfield earthquake sequence and Parkfield prediction experiment , Tectonophysics , 270 , 207 – 219 .
Kagan Y.Y. 1997 . Seismic moment-frequency relation for shallow earthquakes: Regional comparison , J. geophys. Res. , 102 , 2835 – 2852 .
Kagan Y.Y. Jackson D.D. 1996 . Statistical tests of VAN earthquake predictions: Comments and reflections , Geophys. Res. Lett. , 23 , 1433 – 1436 .
Kagan Y.Y. Knopoff L. 1987 . Statistical short-term earthquake prediction , Science , 236 , 1563 – 1567 .
Kanamori H. 1970 . Recent developments in earthquake prediction research in Japan , Tectonophysics , 9 , 291 – 300 .
Kanamori H. 1996 . Initiation process of earthquakes and its implications for seismic hazard reduction strategy , Proc. Nat. Acad. Sci. USA , 93 , 3726 – 3731 .
Kanamori H. Fuis G. 1976 . Variation of P-wave velocity before and after the Galway Lake Earthquake ( M L = 5.2) and the Goat Moutain earthquakes ( M L = 4.7,4.7), 1975, in the Mojave Desert, California , Bull. seism. Soc. Am. , 66 , 2017 – 2037 .
Kanamori H. Satake K. 1990 . Broadband study of the 1989 Loma Prieta earthquake , Geophys. Res. Lett. , 17 , 1179 – 1182 .
Katzoff J.A. 1985 . Earthquake watch to be discussed , Eos. Trans. Am. geophys. Un. , 66 , 773 .
Kaula W.M. 1974 . The next California earthquake (book review) . Science , 186 , 728 – 729 .
Keilis-Borok V.I. 1990 . Special issue: Intermediate-term earthquake prediction: models, algorithms, world-wide tests , Phys. Earth planet. Inter. , 61 , 1 – 139 .
Kerr R.A. 1978 . Earthquakes: prediction proving elusive , Science , 200 , 419 – 421 .
Kerr R.A. 1979 . Prospects for earthquake prediction wane , Science , 206 , 542 – 545 .
Kerr R.A. 1979 . Earthquake prediction: Mexican quake shows one way to look for the big ones , Science , 203 , 860 – 862 .
Kerr R.A. 1980 . Quake prediction by animals gaining respect , Science , 208 , 695 – 696 .
Kerr R.A. 1981 . Palmdale bulge doubts now taken seriously , Science , 214 , 1331 – 1333 .
Kerr R.A. 1981 . Prediction of huge Peruvian quakes quashed , Science , 211 , 808 – 809 .
Kerr R.A. 1981 . Earthquake prediction retracted , Science , 213 , 527 .
Kerr R.A. 1984 . Stalking the next Parkfield earthquake , Science , 223 , 36 – 38 .
Kerr R.A. 1985 . Earthquake forecast endorsed , Science , 228 , 311 .
Kerr R.A. 1985 . Prospects for short-term earthquake prediction , Science , 227 , 42 .
Kerr R.A. 1986 . Parkfield earthquake looks to be on schedule , Science , 231 , 116 .
Kerr R.A. 1986 . Quake prediction under way in earnest , Science , 233 , 520 .
Kerr R.A. 1988 . Waiting for Parkfield—No precursors recognized , Science , 239 , 145 – 146 .
Kerr R.A. 1990 . Signs of the Parkfield quake ?, Science , 249 , 1502 .
Kerr R.A. 1990 . Earthquake—or earthquack , Science , 250 , 511 .
Kerr R.A. 1991 . The lessons of Dr. Browning , Science , 253 , 622 – 623 .
Kerr R.A. 1992 . Seismologists issue a no-win earthquake warning , Science , 258 , 742 – 743 .
Kerr R.A. 1993 . Parkfield quakes skip a beat , Science , 259 , 1120 – 1122 .
Kerr R.A. 1995 . Quake prediction tool gains ground , Science , 270 , 911 – 912 .
Kerr R.A. 1997 . Listening to silent earthquakes , Science , 276 , 1792 – 1793 .
King C.-Y. 1969 . Scismic efficiency , J. geophys. Res. , 74 , 1702 – 1703 .
King C.-Y. (ed.) 1980 . Special section: Geochemical measurements pertinent to earthquake prediction , J. geophys. Res. , 85 , 3051 – 3121 .
King C.-Y. (ed.) 1981 . A special collection of reports on earthquake prediction: Hydrologic and geochemical studies , Geophys. Res. Lett. , 8 , 421 – 484 .
King C.-Y. 1983 . Electromagnetic emissions before earthquakes , Nature , 301 , 377 .
King C.-Y. 1984 . Evaluating hydrological and geochemical anomalies , Nature , 312 , 501 .
King C.-Y. (ed.) 1984 . Special issue: Earthquake hydrology and chemistry , Pure appl. Geophys. , 122 , 141 – 618 .
King C.-Y. (ed.) 1986 . Special section: Gas geochemistry of volcanism, earthquakes, resource exploration, and Earth's interior , J. geophys. Res. , 91 , 12 157 – 12 388 .
King C.-Y. Koizumi N. Kitagawa Y. 1995 . Hydrogeochemical anomalies and the 1995 Kobe earthquake , Science , 269 , 38 – 39 .
Kisslinger C. 1974 . Earthquake prediction , Physics Today , 27 ( 3 ), 36 – 42 .
Kisslinger C. 1976 . The Earthquake Disaster Mitigation Act , Eos. Trans. Am. geophys. Un. , 57 , 437 – 440 .
Kisslinger C. 1978 . Reply , Eos. Trans. Am. geophys. Un. , 59 , 2 .
Kisslinger C. 1983 . Book review of Earthquake Prediction Techniques: Their Application in Japan , edited by Asada T. , Eos. Trans. Am. geophys. Un. , 64 , 105 – 106 .
Kisslinger C. 1984 . Practical approaches to earthquake prediction and warning , Eos. Trans. Am. geophys. Un. , 65 , 398 .
Kisslinger C. 1989 . Portents and predictions , Nature , 339 , 337 – 338 .
Kisslinger C. Rikitake T. 1974 . U.S.-Japan seminar on earthquake prediction and control , Eos. Trans. Am. geophys. Un. , 55 , 9 – 15 .
Kisslinger C. Wyss M. 1975 . Earthquake prediction , Rev. Geophys. Space Phys. , 13 , 298 – 300 , 316 – 319 .
Kisslinger C. Rikitake T. (eds), 1985 . Special issue: Proceedings of symposium on Practical Approaches to Earthquake Prediction and Warning , Earthq. Predict. Res. , 3 , 203 – 685 .
Kisslinger C. Mikumo T. Kanamori H. 1988 . U.S.-Japan quake prediction research , Eos. Trans. Am. geophys. Un. , 69 , 1672 – 1674 .
Kittl P. Diaz G. Martinez V. 1993 . Principles and the uncertainty principles of the probabilistic strength of materials and their applications to seismology , ASME Appl. Mech. Rev. , 46 , S327 – S333 .
Knopoff L. 1996 . Earthquake prediction—the scientific challenge , Proc. Nat. Acad. Sci. USA , 93 , 3719 – 3720 .
Knopoff L. Aki K. Allen C.R. Rice J.R. Sykes L.R. (eds), 1996 . Earthquake Prediction: The Scientific Challenge , Colloquium Proceedings , Proc. Nat. Acad. Sci. USA , 93 , 3719 – 3837 .
Koizumi N. 1997 . Review of geochemical research for earthquake prediction , Shizen Saigai Kagaku. 16 ( 1 ), 41 – 60 (in Japanese).
Kossobokov V.G. Healy J.H. Dewey J.W. 1997 . Testing an earthquake prediction algorithm . Pure appl. Geophys. , 149 , 219 – 232 .
Kovach R.L. Nur A. (eds), 1973 . Proc. Conference on Tectonic Problems of the San Andreas Fault System , Stanford University Publ. Geol. Sci. , vol. 13 , Stanford, CA.
Kulhánek O. (ed.) 1988 . Special issue: Seismic source physics and earthquake prediction research , Tectonophysics , 152 , 165 – 334 .
Landsberg H. 1935 . The problem of earthquake prediction , Science , 82 , 37 .
Langbein J. 1991 . Earthquake explanations , Nature , 349 , 287 .
Langbein J. 1992 . The October 1992 Parkfield, California, earthquake prediction , Earthq. Volc. , 23 , 160 – 169 .
Langbein J. 1993 . Parkfield: First short-term warning , Eos. Trans. Am. geophys. Un. , 74 , 152 – 153 .
Langbein J.O. Burford R.O. Slater L.E. 1990 . Variations in fault slip and strain accumulation at Parkfield, California: Initial results using two-color geodimeter measurements, 1984–1988 , J. geophys. Res. , 95 , 2533 – 2552 .
Langmuir I. 1989 . Pathological science , Physics Today , 42 ( 10 ), 36 – 48 .
Lapwood E.R. 1977 . Earthquake prediction , Nature , 266 , 220 – 221 .
Lay T. Wallace T.C. 1995 . Modern Global Seismology , Academic , San Diego, CA.
Leary P.C. 1997 . Rock as a critical-point system and the inherent implausibility of reliable earthquake prediction , Geophys. J. Int. , 131 , 451 – 466 (this issue).
Leary P.C. Malin P. 1982 . Millisecond accurate monitoring of seismic traveltimes over 13- and 18-kilometer baselines , J. geophys. Res. , 87 , 6919 – 6930 .
Lerner-Lam A. 1997 . Predictable debate , Seism. Res. Lett. , 68 , 381 – 382 .
Li H. Kerr R.A. 1997 . Warnings precede Chinese temblors , Science , 276 , 526 .
Li H. Mervis J. 1996 . China's campaign to predict quakes , Science , 273 , 1484 – 1486 .
Lighthill J. (ed.) 1996 . A Critical Review of VAN , World Scientific , Singapore.
Linde A.T. Sacks I.S. 1997 . Slow earthquakes, stress redistribution, and fast earthquakes , Eos. Trans. Am. geophys. Un. (Spring Mtg. Suppl. ), 78 , S214 (abstract).
Linde A.T. Gladwin M.T. Johnston M.J.S. 1992 . The Loma Prieta earthquake, 1989 and earth strain tidal amplitudes: an unsuccessful search for associated changes , Geophys. Res. Lett. , 19 , 317 – 320 .
Linde A.T. Gladwin M.T. Johnston M.J.S. Gwyther R.L. Bilham R.G. 1996 . A slow earthquake sequence on the San Andreas Fault , Nature , 383 , 65 – 68 .
Lindh A.G. 1983 . Preliminary assessment of long-term probabilities for large earthquakes along selected fault segments of the San Andreas Fault system in California , U.S. Geological Survey, Open-File Report , 83 – 63 .
Lindh A.G. 1990 . Earthquake prediction comes of age, Technology Review , February/March 1990 issue , 42 – 51 .
Lindh A.G. Lockner D.A. Lee W.H.K. 1978 . Velocity anomalies: alternative explanation , Bull. seism. Soc. Am. , 68 , 721 – 734 .
Lindh A. Evans P. Harsh P. Buhr G. 1979 . The Parkfield prediction experiment , Earthq. Info. Bull. , 11 , 209 – 213 .
Lisowski M. Prescott W.H. Savage J.C. Svarc J.L. 1990 . A possible geodetic anomaly observed prior to the Loma Prieta, California, earthquake , Geophys. Res. Lett. , 17 , 1211 – 1214 .
Logan J.M. 1977 . Animal behaviour and earthquake prediction , Nature , 265 , 404 – 405 .
Lomnitz C. 1994 . Fundamentals of Earthquake Prediction , Wiley , New York, NY.
Lomnitz C. Lomnitz L. 1978 . Tangshan 1976: A case history in earthquake prediction , Nature , 271 , 109 – 111 .
Lubkin G.B. 1973 . Do earthquakes give advance warning signals Physics Today , 26 ( 7 ), 17 – 20 .
Ma Z. et al. , (eds), 1990 . Earthquake Prediction: Nine Major Earthquakes in China (1966–1976) . Springer-Verlag , Berlin.
Macelwane J.B. 1946 . Forecasting earthquakes , Bull. seism. Soc. Am. , 36 , 1 – 4 (reprinted in Geophys. J. Int., 131 , 421 – 422 , this issue).
Main I.G. 1995 . Earthquakes as critical phenomena: implications for probabilistic seismic hazard analysis , Bull. seism. Soc. Am. , 85 , 1299 – 1308 .
Main I.G. 1996 . Statistical physics, seismogenesis, and seismic hazard , Rev. Geophys. , 34 , 433 – 462 .
Main L.G. 1997 . Long odds on prediction , Nature , 385 , 19 – 20 .
Marder M. Fineberg J. 1996 . How things break , Physics Today , 49 ( 9 ), 24 – 29 .
Mark R.K. Tinsley J.C. III Newman E.B. Gilmore T.D. Castle R.O. 1981 . An assessment of the accuracy of the geodetic measurements that define the southern California uplift , J. geophys. Res. , 86 , 2783 – 2808 .
Mark R.K. Gilmore T.D. Castle R.O. 1987 . Evidence of suppression of the unequal refraction error in geodetic leveling , J. geophys. Res. , 92 , 2767 – 2790 .
Markov V. 1988 . Armenians face up to scenes of total devastation , Nature , 336 , 608 – 609 .
Masood E. 1995 . Greek earthquake stirs controversy over claims for prediction method , Nature , 375 , 617 .
Masood E. 1995 . Court charges open split in Greek earthquake experts , Nature , 377 , 375 .
Matsumura S. 1993 . Overestimates of earthquake prediction efficiency in a ‘post-prediction state ’, J. Phys. Earth , 51 , 41 – 43 .
Matsumura S. 1997 . Focal zone of a future Tokai earthquake inferred from the seismicity pattern around the plate interface , Tectonophysics , 273 , 271 – 291 .
Matsu'ura R.S. 1986 . Precursory quiescence and recovery of aftershock activities before some large aftershocks , Bull. Earthq. Res. Inst. Univ. Tokyo , 61 , 1 – 65 .
McEvilly T.V. 1976 . Book review of Earthquake Prediction (by T. Rikitake) , Bull. seism. Soc. Am. , 66 , 1774 .
McEvilly T.V. (ed.) 1982 . Special symposium: Invited papers on earthquake prediction , Bull. seism. Soc. Am. , 72 , S331 – S392 .
McEvilly T.V. Johnson L.R. 1973 . Earthquakes of strike-slip type in central California: Evidence on the question of dilatancy , Science , 182 , 581 – 584 .
McEvilly T.V. Johnson L.R. 1974 . Stability of P and S velocities from central California quarry blasts , Bull. seism. Soc. Am. , 64 , 343 – 353 .
McNally K.C. 1982 . Variations in seismicity as a fundamental tool in earthquake prediction , Bull. seism. Soc. Am. , 72 , S351 – S366 .
Medawar P.B. 1979 . Advice to a Young Scientist . Harper & Row , New York, NY.
Meeus J. 1975 . Comments on The Jupiter Effect , Icarus , 26 , 257 – 267 .
Meeus J. 1975 . Reply to Gribben and Plagemann , Icarus , 26 , 270 .
Meeus J. 1976 . Sunspots and earthquakes , Physics Today , 29 ( 6 ), 11 .
Mei S. 1992 . Progress in earthquake prediction in China during the 80's . J. Earthq. Pred. , 1 , 43 – 57 .
Michael A.J. 1996 . The evaluation of VLF guided waves as possible earthquake precursors , U.S. Geological Survey Open-file report , 96 – 67 .
Michael A.J. 1997 . Testing prediction methods: Earthquake clustering versus the Poisson model , Geophys. Res. Lett. , 24 , 1891 – 1894 .
Michael A.J. Langbein J. 1993 . Earthquake prediction lessons from Parkfield experiment , Eos. Trans. Am. geophys. Un. , 74 , 145 – 155 .
Mikumo T. Aki K. Ohnaka M. Ruff L.J. Spudich P.K.P. (eds), 1992 . Special issue of papers from Intl. Symp. on Earthquake source physics and earthquake precursors , Tectonophysics , 211 , 1 – 344 .
Mileti D.S. Fitzpatrick C. 1993 . The Great Earthquake Experiment: Risk Communication and Public Action , Westview , Boulder, CO.
Miller S.A. 1996 . Fluid-mediated influence of adjacent thrusting on the seismic cycle at Parkfield , Nature , 382 , 799 – 802 .
Milne J. 1880 . Seismic science in Japan , Trans. seism. Soc. Jpn. 1 , 3 – 33 .
Milne J. 1911 . The observatory at Messina , Nature , 85 , 515 – 516 .
Milne J. 1911 . Earthquakes and luminous phenomena , Nature , 87 , 16 .
Mogi K. 1970 . Recent horizontal deformation of the Earth's crust and tectonic activity in Japan (1) , Bull. Earthq. Res. Inst. Univ. Tokio. 48 , 413 – 430 .
Mogi K. 1981 . Earthquake prediction program in Japan , in Earthquake Prediction: An International Review , eds Simpson D.W. Richards P.G. , Ewing Monograph Series 4 , pp. 635 – 666 . Am. Geophys. Un. , Washington, DC.
Mogi K. 1984 . Temporal variation of crustal deformation during the days preceding a thrust-type great earthquake—The 1944 Tonankai earthquake of Magnitude 8.1, Japan , Pure appl. Geophys. , 122 , 765 – 780 .
Mogi K. 1985 . Earthquake Prediction , Academic , Orlando, FL.
Mogi K. 1986 . Recent earthquake prediction research in Japan , Science , 233 , 324 – 330 .
Mogi K. 1992 . Earthquake prediction research in Japan , in Earthquake Prediction—Proceedings of the International School of Solid Earth Geophysics, 5th course , pp. 11 – 31 , eds Dragoni M. Boschi E. Il Cigno Galileo Galilei , Rome.
Mogi K. 1995 . Earthquake prediction research in Japan . J. Phys. Earth. 43 , 533 – 561 .
Mogi K. 1996 . Earthquake policy rests on shaky ground , Views from Japan , 1 ( 9 ), Foreign Press Center , Tokyo, Oct. 1996 (translation and abridgement of article in This is Yomiuri. Oct. 1996).
Monastersky R. 1997 . The overdue quake , Science News. 152 , 8 – 9 .
Mori J. Kanamori H. 1996 . Initial rupture of earthquakes in the 1995 Ridgecrest, California, earthquake sequence , Geophys. Res. Lett. , 23 , 2437 – 2440 .
Mulargia F. 1997 . Retrospective validation of time association , Geophys. J. Int. , 131 , 500 – 504 (this issue).
Mulargia F. Gasperini P. 1992 . Evaluating the statistical validity beyond chance of VAN earthquake precursors , Geophys. J. Int. , 111 , 32 – 44 .
Mulargia F. Marzocchi W. Gasperini P. 1996 . Rebuttal to replies I and II by Varotsos et al. , Geophys. Res. Lett. , 23 , 1339 – 1340 .
Mulargia F. Marzocchi W. Gasperini P. 1996 . Re-Rebuttal to the reply of Varotsos et al. , Geophys. Res. Lett. , 23 , 1343 – 1344 .
Myachkin V.I. Kostrov B.V. Sobolev G.A. Shamina O.G. 1986 . Fundamentals of physics of the earthquake focus forerunners , pp. 1 – 24 , in Physics of the Earthquake Focus , ed. Sadovskii M.A. Balkema A.A. Rotterdam (Translation of Fizika Ochaga Zemletryaseniya , Nauka , Moscow, 1975).
NASDA 1997 . Abstracts, International Workshop on Seismo Electronics . 35 March 1997, University of Electro-Communications , Tokyo, Japan.
Nersesov L. 1970 . Earthquake prognostication in the Soviet Union , Bull. New Zealand Soc. Earthq. Eng. , 3 , 108 – 119 .
Nishida N. 1989 . Public opinion regarding earthquake prediction . Seism. Soc. Jpn Newsletter , 1 ( 1 ), 58 – 59 (in Japanese).
Nishimura E. Hosoyama K. 1953 . On tilting motion of ground observed before and after the occurrence of an earthquake , Trans. Am. geophys. Un. , 34 , 597 – 599 .
Norman C. 1977 . Warning in time , Nature , 268 , 684 .
Normile D. 1994 . Japan holds firm to shaky science , Science , 264 , 1656 – 1658 .
Normile D. 1996 . Chair quits Japan panel in protest , Science , 271 , 1799 .
Normile D. 1997 . Earthquake prediction: Report slams Japanese program , Science , 275 , 1870 .
Nur A. 1972 . Dilatancy, pore fluids, and premonitory variations of t s /t p traveltimes , Bull. seism. Soc. Am. , 62 , 1217 – 1222 .
Office of Technology Assessment 1995 . Reducing Earthquake Losses . Report OTA-ETI-623. Sept. 1995. Congress of the United States , Washington, DC.
Ogata Y. Utsu T. Katsura K. 1996 . Statistical discrimination of foreshocks from other earthquake clusters , Geophys. J. Int. , 127 , 17 – 30 .
Ohta H. Abe K. 1977 . Responses to earthquake prediction in Kawasaki City, Japan, in 1974 , J. Phys. Earth , 25 , S273 – S282 .
Ohtake M. Matumoto T. Latham G.V. 1977 . Seismicity gap near Oaxaca, southern Mexico as a probable precursor to a large earthquake , Pure appl. Geophys. , 115 , 375 – 385 .
Ohtake M. Matumoto T. Latham G.V. 1981 . Evaluation of the forecast of the 1978. Oaxaca, southern Mexico earthquake based on a precursory seismic quiescence , in Earthquake Prediction: An International Review , eds Simpson D.W. Richards P.G. , Ewing Monograph Series 4 , pp. 53 – 61 , Am. Geophys. Un. , Washington, DC.
Okada Y. 1984 . First results from Japanese network for earthquake prediction , Nature , 312 , 500 – 501 .
Oliver J. 1964 . Earthquake prediction , Science , 144 , 1364 – 1365 .
Oliver J. 1966 . Earthquake prediction , Science , 153 , 1024 – 1025 .
Olson R.S. Podesta B. Nigg J.M. 1989 . The Politics of Earthquake Prediction , Princeton University Press , Princeton, NJ.
Otsuka M. 1972 . A chain-reaction-type source model as a tool to interpret the magnitude-frequency relation of earthquakes , J. Phys. Earth , 20 , 35 – 45 .
Otsuka M. 1972 . A simulation of earthquake recurrence , Phys. Earth planet. Inter. , 6 , 311 – 315 .
Pakiser L.C. Eaton J.P. Healy J.H. Raleigh C.B. 1969 . Earthquake prediction and control , Science , 166 , 1467 – 1474 .
Papanikolaou D. et al. 1997 . Roundtable discussion on earthquake prediction; conclusions—recommendations , Geophysical Newsletter , No. 18 , Hellenic Geophysical Union , Feb. 1997.
Park S.K. 1992 . Workshop on low-frequency electrical precursors to earthquakes , Eos. Trans. Am. geophys. Un. , 73 , 491 – 492 .
Park S.K. 1996 . Precursors to earthquakes: Seismoelectromagnetic signals , Surv. Geophys. , 17 , 493 – 516 .
Park S.K. Johnston M.J.S. Madden T.R. Morgan F.D. Morrison H.F. 1993 . Electromagnetic precursors to earthquakes in the ULF band: a review of observations and mechanisms , Rev. Geophys. , 31 , 117 – 132 .
Parrot M. 1995 . Electromagnetic noise due to earthquakes , in Handbook of Atmospheric Electrodynamics , vol. II , pp. 95 – 116 , ed. Volland H. CRC Press , Boca Raton, FL.
Parrot M. Fraser-Smith A.C. Molchanov O.A. Yoshmo T. 1995 . Electromagnetic effects associated with earthquakes and volcanic eruptions , Eos. Trans. Am. geophys. Un. , 76 , 223 .
Poley C.M. Lindh A.G. Bakun W.H. Schulz S.S. 1987 . Temporal changes in microseismicity and creep near Parkfield, California , Nature , 327 , 134 – 137 .
Press F. 1975 . Earthquake prediction , Sci. Am. , 232 ( 5 ), 14 – 23 .
Press F. 1975 . Earthquake prediction , Eos. Trans. Am. geophys. Un. , 56 , 275 (reprinted from New York Times ).
Press F. 1975 . Plate tectonics and earthquake prediction: Contrasting approaches in China and the United States , Bull. Am. Acad. Arts Sci. , 28 , 14 – 27 .
Press F. 1976 . Haicheng and Los Angeles: A tale of two cities , Eos. Trans. Am. geophys. Un. , 57 , 435 – 436 .
Press F. Brace W.F. 1966 . Earthquake prediction , Science , 152 , 1575 – 1584 .
Press F. (chairman) et al. 1965 . Earthquake Prediction: A proposal for a ten year program of research, Ad Hoc Panel on Earthquake Prediction, prepared for Office of Science and Technology. Washington, Sept. 1965 .
Press F. et al. 1975 . Earthquake research in China , Eos. Trans. Am. geophys. Un. , 56 , 838 – 881 .
Quan Y.-D. 1988 . The Haicheng, Liaoning Province, earthquake of M7.3 of February 4, 1975 , in Earthquake Cases in China (1966–1975) , pp. 189 – 210 , ed. Zhang Z.-C. Seismological Press , Beiging (in Chinese).
Raleigh B. 1982 . A strategy for short-term prediction of an earthquake , Bull. seism. Soc. Am. 72 , S337 – S342 .
Raleigh C.B. Healy J.H. Bredehoeft J.D. 1976 . An experiment in earthquake control at Rangely, Colorado , Science , 191 , 1230 – 1237 .
Raleigh C.B. et al. 1977 . Prediction of the Haicheng earthquake , Eos. Trans. Am. geophys. Un. , 58 , 236 – 272 .
Raleigh C.B. Sich K. Sykes L.R. Anderson D.L. 1982 . Forecasting southern California earthquakes , Science , 217 , 1097 – 1104 .
Rao B.R. 1985 . New trends in earthquake prediction , Eos. Trans. Am. geophys. Un. , 66 , 796 – 797 .
Reasenberg P. 1978 . Unusual animal behaviour before earthquakes , Earthq. Info. Bull. , 10 , 42 – 50 .
Reasenberg P. 1996 . Tests of SEISMOLAP-based methods for earthquake prediction in California , Eos. Trans. Am. geophys. Un. , 77 , ( Fall Meeting suppl. ), F458 (abstract).
Reasenberg P.A. Matthews M.V. 1988 . Precursory seismic quiescence: a preliminary assessment of the hypothesis , Pure appl. Geophys. , 126 , 373 – 406 .
Reid H.F. 1910 . The California Earthquake of April 18, 1906, vol. II, The Mechanics of the Earthquake . Rest State Earthquake Investigation Commission, Carnegie Institution of Washington , Washington, DC.
Reid H.F. 1920 . The problems of seismology , Proc. Nat. Acad. Sci. U.S. , 6 , 555 – 561 ; reprinted in Trans. Am. Geophys. Un., 1 , Reprint and circular series No. 11.
Reilinger R. 1980 . Elevation changes near the San Gabriel fault, southern California . Geophus. Res. Lett. , 7 , 1017 – 1019 .
Reilinger R. Brown L. 1981 . Neotectonic deformation, near-surface movements and systematic errors in U.S. releveling measurements: implications for earthquake prediction , in Earthquake Prediction: An International Review , eds Simpson D.W. Richards P.G. Ewing Monograph Series 4 , pp. 422 – 440 . Am. Geophys. Un. , Washington, DC.
Rhoades D.A. Evison F.F. 1989 . On the reliability of precursors , Phys. Earth planet. Inter. , 58 , 137 – 140 .
Rhoades D.A. Evison F.F. 1989 . Time-variable factors in earthquake hazard . Tectonophysics , 167 , 201 – 210 .
Rhoades D.A. Evison F.F. 1996 . The VAN earthquake predictions , Geophys. Res. Lett. , 23 , 1371 – 1374 .
Richman B.T. 1981 . Prediction of Peru quakes rejected , Eos. Trans Am. geophys. Un. , 62 , 129 .
Richman B.T. 1981 . Peru earthquake prediction update , Eos. Trans Am. geophys. Un. , 62 , 618 .
Richter C.F. 1958 . Elementary Seismology , W. H. Freeman , San Francisco, CA.
Richter C.F. 1964 . Discussion of paper by V.I. Keylis-Borok and L.N. Malinovskaya , J. geophys. Res. , 69 , 3025 .
Richter C.F. 1977 . Acceptance of the Medal of the Seismological Society of America . Bull. seism. Soc. Am. , 67 , 1244 – 1247 .
Rikitake T. 1966 . A five-year plan for earthquake prediction research in Japan . Tectonophysics , 3 , 1 – 15 .
Rikitake T. (ed.) 1968 . Special issue: Earthquake prediction , Tectonophysics , 6 , 7 – 87 .
Rikitake T. (ed.) 1970 . Special issue: Earthquake mechanics , Tectonophysics , 9 , 97 – 300 .
Rikitake T. 1972 . Problems of predicting earthquakes , Nature , 240 , 202 – 204 .
Rikitake T. (ed.) 1974 . Special issue: Focal processes and the prediction of earthquakes , Tectonophysics , 23 , 219 – 318 .
Rikitake T. 1974 . Japanese national program on earthquake prediction , Tectonophysics , 23 , 225 – 236 .
Rikitake T. 1975 . Earthquake precursors , Bull. seism. Soc. Am. , 65 , 1133 – 1162 .
Rikitake T. 1976 . Earthquake Prediction , Elsevier , Amsterdam.
Rikitake T. 1979 . The large-scale earthquake countermeasures act and the earthquake prediction council in Japan , Eos. Trans. Am. geophys. Un. , 60 , 553 – 555 .
Rikitake T. (ed.) 1981 . Current Research in Earthquake Prediction I . D. Reidel , Dordrecht/CAPJ, Tokyo.
Rikitake T. 1981 . Practical approach to earthquake prediction and warning , in Current Research in Earthquake Prediction I , ed. Rikitake T. pp. 1 – 80 , D. Reidel , Dordrecht/CAPJ, Tokyo.
Rikitake T. (ed.) 1984 . Earthquake Prediction: Proceedings of the International Symposium on Earthquake Prediction , Terrapub , Tokyo; Unesco , Paris.
Rikitake T. 1986 . Earthquake prediction in the heart of Japan , Earthq. Pred. Res. , 4 , 213 – 486 .
Rikitake T. 1987 . Earthquake precursors in Japan: Precursor time and detectability , Tectonophysics , 136 , 265 – 282 .
Rikitake T. 1988 . Earthquake prediction: An empirical approach , Tectonophysics , 148 , 195 – 210 .
Rikitake T. 1991 . Book review of Earthquake Prediction—Present Status , ed. Guha S.K. Patwardhan A.M. (eds), Tectonophysics , 200 , 333 – 334 .
Robinson R. Wesson R.L. Ellsworth W.L. 1974 . Variation of P-wave velocity before the Bear Valley, California, earthquake of 24 February 1972 , Science , 184 , 1281 – 1283 .
Roeloffs E. Langbein J. 1994 . The earthquake prediction experiment at Parkfield. California , Rev. Geophys. , 32 , 315 – 336 .
Roeloffs E. Quilty E. 1997 . Case 21: Water level and strain changes preceding and following the August 4, 1985 Kettleman Hills, California, earthquake . Pure appl. Geophys. , 149 , 21 – 60 .
Roeloffs E.A. Burford S.S. Riley F.S. Records A.W. 1989 . Hydrologic effects on water level changes associated with episodic fault creep near Parkfield, California , J. geophys. Res. , 94 , 12 387 – 12 402 .
Rundle J.B. McNutt M. 1981 . Southern California uplift—Is it or isn't it ?, Eos. Trans. Am. geophys. Un. , 62 , 97 .
Russ D.P. Johnson L.E. 1985 . Cooperative earthquake research between the United States and the People's Republic of China , Eos. Trans. Am. geophys. Un. , 66 , 763 – 765 .
Sadovsky M.A. Nersesov I.L. 1974 . Forecasts of earthquakes on the basis of complex [geophysical] features , Tectonophysics , 23 , 247 – 255 .
Sadovsky M.A. Nersesov I.L. Nigmatullaev L.A. Latynina L.A. Lukk A.A. Semenov A.N. Simbireva I.G. Ulomov V.I. 1972 . The processes preceding strong earthquakes in some regions of middle Asia , Tectonophysics , 14 , 295 – 307 .
Sassa K. Nishimura E. 1951 . On precursory phenomena forerunning earthquakes . Trans. Am. Geophys. Un. , 32 , 1 – 6 .
Savage J.C. 1991 . Criticism of some forecasts of the National Earthquake Prediction Evaluation Council , Bull. seism. Soc. Am. , 81 , 862 – 881 .
Savage J.C. 1992 . The uncertainty in earthquake conditional probabilities , Geophys. Res. Lett. , 19 , 709 – 712 .
Savage J.C. 1993 . The Parkfield prediction fallacy , Bull. seism. Soc. Am. , 83 , 1 – 6 .
Savage J.C. 1996 . No: The L.A. array is not ready for prime time , Eos. Trans. Am. geophys. Un. , 77 , 419 – 427 .
Savage J.C. Prescott W.H. 1979 . Geodimeter measurements of strain during the southern California uplift , J. geophys. Res. , 84 , 171 – 177 .
Savage J.C. Prescott W.H. Lisowski M. King N.E. 1981 . Strain on the San Andreas Fault near Palmdale, California: Rapid, aseismic change , Science , 211 , 56 – 58 .
Savage J.C. Prescott W.H. Losowski M. King N.E. 1981 . Strain accumulation in southern California, 1973–1980 , J. geophys. Res. , 86 , 6991 – 7001 .
Savarenskij E.F. Nersesov I.L. 1978 . Earthquake prediction , in The Assessment and Mitigation of Earthquake Risk , pp. 66 – 90 , UNESCO , Paris.
Savarensky E.F. 1968 . On the prediction of earthquakes , Tectonophysics , 6 , 17 – 27 .
Savarensky E.F. (ed.) 1972 . Special section: Forerunners of strong earthquakes , Tectonophysics , 14 , 177 – 338 .
Schaefer E. Swinbanks D. 1990 . Betting on an inexact science , Nature , 348 , 273 .
Scholz C.H. 1977 . A physical interpretation of the Haicheng earthquake prediction , Nature , 267 , 121 – 124 .
Scholz C.H. 1985 . The Black Mountain asperity: Seismic hazard of the southern San Francisco peninsula, California , Geophys. Res. Lett. , 12 , 717 – 719 .
Scholz C.H. 1997 . Whatever happened to earthquake prediction , Geotimes , 42 ( 3 ), 16 – 19 .
Scholz C.H. Sykes L.R. Aggarwal Y.P. 1973 . Earthquake prediction: ***a physical basis , Science , 181 , 803 – 810 .
Segall P. 1996 . Earthquakes: slow down for safety , Nature , 383 , 21 – 22 .
Segall P. Du Y. 1993 . How similar were the 1934 and 1996 Parkfield earthquakes ?, J. geophys. Res. , 98 , 4527 – 4538 .
Segall P. Harris R. 1986 . Slip deficit on the San Andreas fault at Parkfield, California, as revealed by inversion of geodetic data , Science , 233 , 1409 – 1413 .
Segall P. Harris R. 1987 . Earthquake deformation cycle on the San Andreas fault near Parkfield, California , J. geophys. Res. , 92 , 10 511 – 10 525 .
Segall P. Harris R. 1989 . Comment on: ‘Geodetic evidence for seismic potential at Parkfield, California’ by L.-Y. Sung and D.D. Jackson . Geophys. Res. Lett. , 16 , 101 – 104 .
Segall P. Lisowski M. 1990 . Surface displacements in the 1906 San Francisco and 1989 Loma Prieta earthquakes , Science , 250 , 1241 – 1244 .
Semenov A.M. 1969 . Variations in the travel-time of transverse and longitudinal waves before violent earthquakes , Izvestia, Acad. Sci. USSR Phys. Solid Earth (Engl. Transl.) , No. 4 , 245 – 248 .
Shapley D. 1976 . Earthquakes: Los Angeles prediction suggests faults in federal policy , Science , 192 , 535 – 537 .
Shapley D. 1976 . Chinese earthquakes: The Maoist approach to seismology , Science , 193 , 656 – 657 .
Shaw R.H. Smietana P.J. Jr 1983 . Temperature stratification and refraction errors in geodetic leveling , J. geophys. Res. , 88 , 10 485 – 10 494 .
Shearer C.F. 1985 . Minutes of the National Earthquake Prediction Evaluation Council, November 16–17, 1984 , USGS Open File Report , 85 – 201 .
Shearer C.F. 1985 . Minutes of the National Earthquake Prediction Evaluation Council, March 29–30, 1985 , USGS Open File Report , 85 – 507 .
Shearer C.F. 1986 . National Earthquake Prediction Evaluation Council Special Report I. Workshop on special study areas in southern California, San Diego, CA, Feb. 28-Mar. 2 1985 , USGS Open File Rept. , 86 – 580 .
Shimazaki K. Stuart W. (eds), 1984 . Special issue of papers presented at the earthquake prediction session of the 22nd general assembly of the Intl. Union of Geodesy & Geophysics, Hamburg, Germany, Aug. 1983 , Pure appl.*** Geophys. , 122 , 763 – 997 .
Shimazaki K. Wakita H. (eds), 1986 . Proceedings of the Japan-China symposium on earthquake prediction , J. Phys. Earth , 34 , S1 – S291 .
Silver P.G. Valette-Silver N.J. 1992 . Detection of hydrothermal pr***ecursors to large northern California earthquakes , Science , 257 , 1363 – 1368 .
Silver P.G. Valette-Silver N.J. Kolbek O. 1993 . Detection of hydrothermal precursors to large northern California earthquakes , in The Loma Prieta, California, Earthquake of October 17, 1989—Preseismic Observations , ed. Johnston M. J. S. U.S. Geological Survey Professional Paper 1550-C, pp. C73 – C80 , Washington, DC.
Silver P. Wakita H. 1996 . A search for earthquake precursors , Science , 273 , 77 – 78 .
Simpson D.W. 1979 . Soviet prediction of a major earthquake . Earthq. Info. Bull. , 11 , 234 – 235 .
Simpson D.W. 1980 . Earthquake prediction , Nature , 286 , 445 – 446 .
Simpson D.W. Richards P.G. , eds 1981 . Earthquake Prediction: An International Review Ewing Monograph Series 4 , Am. Geophys. Un. , Washington, DC.
Smith P.J. 1974 . Earthquakes predicted , Nature , 252 , 9 – 11 .
Smith P.J. 1974 . Dilatancy, without fluid flow ?, Nature , 252 , 444 .
Smith P.J. 1975 . Getting closer to prediction , Nature , 253 , 593 .
Smith P.J. 1975 . Crack theory developed , Nature , 253 , 593 – 594 .
Smith P.J. 1975 . Successful quake prediction made , Nature , 255 , 282 .
Smith P.J. 1976 . Radon to predict earthquakes , Nature , 261 , 97 .
Sornette D. Knopoff L. 1997 . The paradox of the expected time until the next earthquake , Bull. seism. Soc. Am. , 87 , 789 – 798 .
Sornette D. Knopoff L. Kagan Y.Y. Vanneste C. 1996 . Rank-ordering statistics of extreme events: Application to the distribution of large earthquakes , J. geophys. Res. , 101 , 13 883 – 13 893 .
Spall H. 1977 . An interview with Robert M. Hamilton , Earthq. Info. Bull.*** 9 ( 6 ), 7 – 9 .
Spall H. 1979 . Earthquake research in the Soviet Union , Earthq. Info. Bull.*** 11 , 22 – 25 .
Spall H. 1980 . Earthquake prediction in the Soviet Union—An interview with I.L. Nersesov , Earthq. Info. Bull. , 12 , 60 – 63 .
Spence W. Pakiser L.C. 1978 . Conference report: Toward earthquake prediction on the global scale , Eos. Trans. Am. geophys. Un. , 59 , 36 – 42 .
Spence W. Hermann R.B. Johnston A.C. Reagor G. 1993 . Responses to Iben Browning's Prediction of a 1990 New Madrid, Missouri, Earthquake , U.S. Geological Survey Circular , 1083 , U.S. Government Printing Office , Washington, DC.
Spudich P. 1996 . Synopsis , in The Loma Prieta, California Earthquake of October 17, 1989—Main-shock Characteristics , U.S. Geological Survey Professional Paper 1550-A, pp. A1 – A7 .
Stacey F.D. 1963 . Seismo-magnetic effect and the possibility of foreca***sting earthquakes , Nature , 200 , 1083 – 1085 .
Stark P.B. 1996 . A few statistical considerations for ascribing statistical significance to earthquake predictions , Geophys. Res. Lett. , 23 , 1399 – 1402 .
Stark P.B. 1997 . Earthquake prediction: the null hypothesis , Geophys. J. Int. , 131 , 495 – 499 (this issue).
Stavrakakis G.N. Drakopoulos J. Latoussakis J. 1990 . Evaluation of the correlation of SES with earthquakes in Greece-time period: 1987–1989. Publ. no 1, Natl. Observ, Athens .
Stein R.S. 1981 . Discrimination of tectonic displacement from slope-dependent errors in geodetic leveling from southern California, 1953–1979 , in Earthquake Prediction: An International Review eds Simpson D.W. Richards P.G. Ewing Monograph Series 4 , pp. 441 – 456 . Am. Geophys. Un. , Washington, DC.
Stein R.S. 1987 . Contemporary plate motions and crustal deformation , Rev. Geophys. , 25 , 855 – 863 .
Stein R.S. 1995 . Characteristic or haphazard? , Nature , 378 , 443 – 444 .
Stein R.S. Whalen C.T. Holdahl S.R. Strange W.E. Thatcher W. 1986 . Saugus-Palmdale, California, field test for refraction error in historical leveling surveys , J. geophys. Res. , 91 , 9031 – 9044 .
Stein R.S. Whalen C.T. Holdahl S.R. Strange W.E. Thatcher W. 1989 . Reply to ‘Comment on “Saugus-Palmdale, California, field test for refraction error in historical leveling surveys” by R.S. Stein, C.T. Whalen, S.R. Holdahl, W. Strange, and W. Thatcher’ by Michael R. Craymer and Peter Vaniíček and Comment on ‘Further analysis of the 1981 southern California field test for leveling refraction’ by M.R. Craymer and P. Vaniíček , J. geophys. Res. , 94 , 7667 – 7672 .
Stewart D.M. 1975 . Is a major earthquake imminent in North Carolina centred near Southport?, Geol. Soc. Am. Southeastern Sect. Mtg., April 9–12, 1975, Memphis, TN , Geol. Soc. Am. Abstracts with Programs , 7 ( 4 ), 540 (abstract).
Stiros S.C. 1995 . Unexpected shock rocks an ‘aseismic’ area , Eos. Trans. Am. geophys. Un. , 76 , 513 – 519 .
Stiros S.C. 1997 . Costs and benefits of earthquake prediction studies in Greece , Geophys. J. Int. , 131 , 478 – 484 (this issue).
Strange W.E. 1981 . The impact of refraction correction on leveling interpretations in southern California , J. geophys. Res. , 86 , 2809 – 2824 .
Strange W.E. 1983 . Reply , J. geophys. Res. , 88 , 2513 – 2519 .
Stuart W.D. 1974 . Diffusionless dilatancy model for earthquake prediction , Geophys. Res. Lett. , 1 , 261 – 264 .
Stuart W.D. 1979 . Strain softening prior to two-dimensional strike slip earthquakes , J. geophys. Res. , 84 , 1063 – 1070 .
Stuart W.D. 1987 . Studies of San Andreas fault , Nature , 327 , 99 – 100 .
Stuart W.D. 1990 . Parkfield slowing down , Nature , 345 , 383 – 384 .
Stuart W.D. 1991 . Seismic quiescence at Parkfield due to detachment faulting , Nature , 349 , 58 – 61 .
Stuart W.D. Aki K. (eds), 1988 . Special issue of papers presented at the international conference, on Physical and observational basis of intermediate-term earthquake prediction , Pure appl. Geophys. , 126 , 175 – 718 .
Stuart W.D. Tullis T.E. 1995 . Fault model for preseismic deformation at Parkfield, California , J. geophys. Res. , 100 , 24 079 – 24 099 .
Stuart W.D. Archuleta R.J. Lindh A.G. 1985 . Forecast model for moderate earthquakes near Parkfield, California , J. geophys. Res. , 90 , 592 – 604 .
Sung L.-Y. Jackson D.D. 1988 . Geodetic evidence of the seismic potential at Parkfield, California , Geophys. Res. Lett. , 15 , 820 – 823 .
Sung L.-Y. Jackson D.D. 1989 . Reply , Geophys. Res. Lett. , 15 , 820 – 823 .
Suzuki Z. 1982 . Earthquake prediction , Ann. Rev. Earth planet. Sci. , 10 , 235 – 256 .
Suzuki Z. 1985 . The fifth 5-year program for earthquake prediction in Japan , Earthq. Predict. Res. , 3 , 207 – 218 .
Suzuki Z. Kisslinger C. (eds), 1977 . U.S.-Japan seminar on earthquake precursors , Eos. Trans. Am. geophys. Un. , 58 , 600 – 603 .
Suzuki Z. Kisslinger C. (eds), 1977 . Proceedings of the U.S.-Japan Seminar on Theoretical and Experimental Investigations of Earthquake Precursors , J. Phys. Earth. 25 , S1 – S296 .
Swinbanks D. 1992 . Trying to shake Japan's faith in forecasts , Nature , 356 , 464 – 465 .
Swinbanks D. 1992 . Without a way to measure their success, Japanese projects are very hard to stop , Nature , 357 , 619 .
Swinbanks D. 1992 . Japanese report shows cracks in predicting earthquakes , Nature , 358 , 361 .
Swinbanks D. 1993 . Earthquake exposes gaps in seismic network , Nature , 364 , 271 .
Swinbanks D. 1993 . Japanese go it alone with earthquake prediction , Nature , 364 , 370 .
Swinbanks D. 1993 . Japan disappoints seekers of foresight , Nature , 366 , 4 .
Swinbanks D. 1994 . Is Japan throwing good money after bad science ?, Nature , 367 , 670 .
Swinbanks D. 1994 . Earthquake ‘forecasters’ face their critics in Japan , Nature , 370 , 9 .
Swinbanks D. 1995 . Kobe disaster divides earthquake researchers , Nature , 373 , 373 .
Swinbanks D. 1995 . Earthquake prediction ‘likely to grow’ after new review , Nature , 374 , 205 .
Swinbanks D. 1995 . Critics attack hasty review of quake prediction efforts , Nature , 374 , 754 .
Swinbanks D. 1997 . Quake panel admits prediction is ‘difficult ’, Nature , 388 , 4 .
Sykes L.R. Nishenko S.P. 1984 . Probabilities of occurrence of large plate rupturing earthquakes for the San Andreas, San Jacinto, and Imperial Faults, California, 1983–2003 , J. geophys. Res. , 89 , 5905 – 5927 .
Sykes L.R. Simpson D.W. Richards P.G. 1981 . Preface to Earthquake Prediction: An International Review , eds Simpson D.W. Richards P.G. Ewing Monograph Series 4 , pp. vi – vii , Am. Geophys. Un. , Washington, DC.
Tang X. 1988 . A General History of Earthquake Studies in China , Science Press , Beijing.
Taubes G. 1993 . Bad Science , Random House , New York, NY.
Taubes G. 1997 . The one that got away ?, Science , 275 , 148 – 151 .
Tazieff H. 1992 . Earthquake Prediction , McGraw-Hill , New York, NY.
Terada T. 1931 . On luminous phenomena accompanying earthquakes . Bull. Earthq. Res. Inst. Tokyo, Imp. Univ. 9 , 225 – 255 .
Thatcher W. 1976 . Episodic strain accumulation in southern California , Science , 194 , 691 – 695 .
Thatcher W. 1979 . Horizontal crustal deformation from historic geodetic measurements in southern California , J. geophys. Res. , 84 , 2351 – 2370 .
Thatcher W. 1981 . Crustal deformation studies and earthquake prediction research , in Earthquake Prediction: An International Review , eds Simpson D.W. Richards P.G. Ewing Monograph Series 4 , pp. 394 – 410 , Am. Geophys. Un. , Washington, DC.
Thatcher W. Marshall G. Lisowski M. 1997 . Resolution of fault slip along the 470-km long rupture of the great 1906 San Francisco earthquake and its implications , J. geophys. Res. , 102 , 5353 – 5367 .
Thurber C.H. 1996 . Creep events preceding small to moderate earthquakes on the San Andreas fault , Nature , 380 , 425 – 428 .
Toppozada T.R. 1985 . Questioning the regular recurrence of characteristic Parkfield events , Eos. Trans. Am. geophys. Un. , 66 , 982 (abstract).
Toppozada T.R. 1992 . Parkfield earthquake history (abstract) , Eos. Trans. Am. geophys. Un. , 73 , 406 .
Tributsch H. 1978 . Do aerosol anomalies precede earthquakes ?, Nature , 276 , 606 – 608 .
Tributsch H. 1982 . When the Snakes Awake , MIT Press , Cambridge, MA.
Tsuboi C. Wadati K. Hagiwara T. 1962 . Prediction of earthquakes: Progress to date and plans for further development . Earthquake Prediction Research Group , Tokyo University (English translation).
Tsunogai U. Wakita H. 1995 . Precursory chemical changes in ground water: Kobe earthquake, Japan , Science , 269 , 61 – 63 .
Tsunogai U. Wakita H. 1996 . Anomalous changes in groundwater chemistry—Possible precursors of the 1995 Hyogo-ken Nanbu earthquake, Japan , J. Phys. Earth , 44 , 381 – 390 .
Tullis T.E. 1994 . Predicting earthquakes and the mechanics of fault slip , Geotimes , 39 ( 7 ), 19 – 21 .
Turcotte D.L. 1991 . Earthquake prediction , Ann. Rev. Earth planet. Sci. , 19 , 263 – 281 .
Turner R.H. 1982 . Media in crisis: blowing hot and cold , Bull. seism. Soc. Am. , 72 , S19 – S28 .
Turner R.H. Nigg J.M. Paz D.H. 1986 . Waiting for Disaster , University of California Press , Berkeley, CA.
Twyman F. 1935 . Prediction of earthquakes , Nature , 135 , 1078 .
UNDRO 1983 . Proc. Seminar on Earthquake Prediction Case Histories (Geneva, 1215 October 1982), convened by Office of U.N. Disaster Relief Co-ordinator and UNESCO , UNDRO/83/33, GE.83-03321.
UNESCO 1979 . Report of panel of experts on the scientific, social, and economic aspects of earthquake prediction , Paris. 912 April 1979. Sc-79/COMF.801/2, report issued 11 June 1979.
US Geological Survey 1976 . Earthquake Prediction—Opportunity to Avert Disaster , US Geological Survey Circular 729 .
US Geological Survey Staff 1990 . The Loma Prieta, California, earthquake: an anticipated event , Science , 247 , 286 – 293 .
Utsu T. 1977 . Possibility of a great earthquake in the Tokai district, Japan , J. Phys. Earth , 25 , S219 – S230 .
Uyeda S. Nagao T. 1997 . Japanese research on electro-magnetic short-term earthquake prediction , Seism. Res. Lett. , 68 , 297 (abstract).
Varotsos P.A. Alexopoulos K.D. 1986 . Thermodynamics of Point Defects and their Relation with Bulk Properties . North-Holland , Amsterdam.
Varotsos P. Kulhánek O. (eds), 1993 . Special issue: Measurements and theoretical models of the earth's electric field variations related to earthquakes , Tectonophysics , 224 , 1 – 288 .
Varotsos P. Lazaridou M. 1991 . Latest aspects of earthquake prediction Greece based on seismic electric signals , Tectonophysics , 188 , 321 – 347 .
Varotsos P. Lazaridou M. Eftaxias K. Antonopoulos G. Makris J. Kopanas J. 1996 . Short-term earthquake prediction in Greece by seismic electric signals , in A Critical Review of VAN , pp. 29 – 76 , ed. Lighthill J. World Scientific , Singapore.
Varotsos P. et al. 1996 . Recent earthquake prediction results in Greece based on the observation of seismic electric signals , Acta Geophysica Polonica , 44 , 301 – 327 .
Varotsos P. Sarlis N. Lazaridou M. Bogris N. 1996 . Statistical evaluation of earthquake prediction results: Comments on the success rate and alarm rate , Acta Geophysica Polonica , 44 , 329 – 347 .
Varotsos P. Eftaxias K. Lazaridou M. 1996 . Reply to ‘A false alarm based on electrical activity recorded at a VAN-station in northern Greece in December 1990.’ by J. Drakopoulos and G. Stavrakakis , Geophys. Res. Lett. , 23 , 1359 – 1362 .
Vere-Jones D. 1976 . A branching model for crack propagation , Pure appl. Geophys. , 114 , 711 – 725 .
Vidale J.E. 1996 . Do big and little earthquakes start differently ?, Science , 271 , 953 – 954 .
Vogel A. (ed.) 1979 . Terrestrial and space techniques in earthquake prediction research , Proc. Int. Workshop on Monitoring Crustal Dynamics in Earthquake Zones, Strasbourg , 29 Aug.-5 Sept. 1978. Friedr. Vieweg & Sohn , Braunschweig.
Wadatsumi K. 1995 . 1519 Precursor Testimonies! , Tokyo Shuppan , Tokyo (in Japanese).
Wakita H. 1981 . Precursory changes in groundwater prior to the 1978 Izu-Oshima-Kinkai earthquake , in Earthquake Prediction: An International Review , eds Simpson D.W. Richards P.G. Ewing Monograph Series 4 , pp. 527 – 532 , Am. Geophys. Un. , Washington, DC.
Wakita H. Nakamura Y. Notsu K. Noguchi M. Asada T. 1980 . Radon anomaly: a possible precursor of the 1978 Izu-Oshima-Kinkai earthquake , Science , 207 , 882 – 883 .
Wakita H. Nakamura Y. Sano Y. 1988 . Short-term and intermediate-term geochemical precursors , Pure appl. Geophys. , 126 , 267 – 278 .
Wakita H. et al. (eds), 1996 . Abstracts, Intl. Symp. on Prediction studies on Earthquake and Volcanic Eruption by Geochemical and Hydrological Methods , 5–8 March 1996. Tokyo, Japan, Bulletin of Laboratory for Earthquake Chemistry, Faculty of Science, University of Tokyo, No. 7 .
Wallace R.E. 1983 . Tangshan. six years later , Earthq. Info. Bull. , 15 , 103 – 107 .
Wallace R.E. Davis J.F. McNally K.C. 1984 . Terms for expressing earthquake potential, prediction, and probability , Bull. seism. Soc. Am. , 74 , 1819 – 1825 .
Walsh J. 1965 . Earthquake prediction: OST panel recommends 10-year program , Science , 150 , 321 – 323 .
Ward P.L. 1979 . Earthquake prediction , Rev. Geophys. Space Phys. , 17 , 343 – 353 .
Warwick J.W. Stoker C. Meyer T.R. 1982 . Radio emission associated with rock fracture: Possible application to the great Chilean earthquake of May 22, 1960 , J. geophys. Res. , 87 , 2851 – 2859 .
Wesnousky S.G. 1996 . Reply to Yan Kagan's comment on ‘The Gutenberg-Richter or characteristic earthquake distribution, which is it ?’, Bull. seism. Soc. Am. , 86 , 286 – 291 .
Wesson R.L. Robinson R. Bufe C.G. Ellsworth W.L. Pfluke J.H. Steppe J.A. Seekins L.C. 1977 . Search for seismic forerunners to earthquakes in central California . Tectonophysics , 42 , 111 – 126 .
Whitcomb J.H. 1976 . Time-dependent V P and V P /V S in an area of the Transverse Ranges of southern California (abstract) , Eos. Trans. Am. geophys. Un. , 57 , 288 .
Whitcomb J.H. Garmany J.D. Anderson D.L. 1973 . Earthquake prediction: Variation of seismic velocities before the San [Fernando] earthquake , Science , 180 , 632 – 635 .
Whiteside L. Habermann R.E. 1989 . The seismic quiescence prior to the 1978 Oaxaca. Mexico earthquake IS NOT a precursor to that earthquake , Eos. Trans. Am. geophys. Un. , 70 , 1232 (abstract).
Wilson E.B. 1952 . An Introduction to Scientific Research , McGraw-Hill , New York, NY (reprinted by Dover , New York, NY, 1990).
Wood H.O. Gutenberg B. 1935 . Earthquake prediction , Science , 82 , 219 – 220 .
Working Group on California Earthquake Probabilities 1988 . Probabilities of large earthquakes occurring in California on the San Andreas Fault , U.S. Geological Survey Open-File Rept , 88 – 398 .
Wu F.T. 1997 . The annual earthquake prediction conference in China (National consultative meeting on seismic tendency) , Pure appl. Geophys. , 149 , 249 – 264 .
Wu K.T. et al. 1978 . Certain characteristics of the Haicheng earthquake (M=7.3) sequence . Chinese Geophys. , 1 , 289 – 308 .
Wuethrich B. 1993 . Waiting for Parkfield to quake , Eos. Trans. Am. geophys. Un. , 74 , 553 – 554 .
Wyatt F.K. Agnew D.C. Gladwin M. 1994 . Continuous measurements of crustal deformation for the 1992 Landers earthquake sequence , Bull. seism. Soc. Am. , 84 , 768 – 779 .
Wyss M. 1975 . Precursors to the Garm earthquake of March 1969 , J. geophys. Res. , 80 , 2926 – 2930 .
Wyss M. (ed.) 1975 . Special issue: Earthquake prediction and rock mechanics , Pure appl. Geophys. , 113 , 1 – 330 .
Wyss M. 1977 . The appearance rate of premonitory uplift , Bull. seism. Soc. Am. , 67 , 1091 – 1098 .
Wyss M. 1977 . Interpretation of the southern California uplift in terms of the dilatancy hypothesis , Nature , 266 , 805 – 808 .
Wyss M. (ed.) 1979 . Special issue: Earthquake prediction and seismicity patterns , Pure appl. Geophys. , 118 , 1079 – 1315 .
Wyss M. 1983 . Earthquake prediction , Rev. Geophys. Space Phys. , 21 , 1291 – 1298 .
Wyss M. 1990 . Changes of mean magnitude of Parkfield seismicity: A part of the precursory process ?, Geophys. Res. Lett. , 17 , 2429 – 2432 .
Wyss M. (ed.) 1991 . Special issue: earthquake prediction , Tectonophysics , 193 , 253 – 410 .
Wyss M. (ed.) 1991 . Evaluation of Proposed Earthquake Precursors . American Geophysical Union , Washington, DC.
Wyss M. 1991 . Increased mean depth of earthquakes at Parkfield , Geophys. Res. Lett. , 18 , 617 – 620 .
Wyss M. 1997 . Cannot earthquakes be predicted ?, Science , 278 , 487 – 488 .
Wyss M. 1997 . Second round of evaluation of proposed earthquake precursors , Pure appl. Geophys. , 149 , 3 – 16 .
Wyss M. 1997 . Case 23: Nomination of precursory seismic quiescence as a significant precursor , Pure appl. Geophys. , 149 , 79 – 113 .
Wyss M. Brune J.N. 1967 . The Alaska earthquake of 28 March 1964: A complex multiple rupture , Bull. seism. Soc. Am. , 57 , 1017 – 1023 .
Wyss M. Dmowska R. , (eds), 1997 . Special issue: Earthquake prediction—state of the art . Pure appl. Geophys. , 149 , 1 – 264 .
Wyss M. Holcomb D.J. 1973 . Earthquake prediction based on station residuals . Nature , 245 , 139 – 140 .
Wyss M. Bodin P. Habermann R.E. 1990 . Seismic quiescence at Parkfield: an independent indication of an imminent earthquake , Nature , 345 , 426 – 428 .
Wyss M. Slater L. Burford R.O. 1990 . Decrease in deformation rate as a possible precursor to the next Parkfield earthquake , Nature , 345 , 428 – 432 .
Wyss M. Habermann R.E. Bodin P. 1992 . Seismic quiescence: a test of the hypothesis and a precursor to the next Parkfield, California, earthquake . Geophys. J. Int. , 110 , 518 – 536 .
Xu S.-X. Wang B.-Q. Jones L.M. Ma X.-F. Shen P.-W. 1982 . The foreshock sequence of Haicheng earthquake and earthquake swarm—the use of foreshock sequences in earthquake prediction , Tectonophysics , 85 , 91 – 105 .
Yoshida A. Furuya I. 1992 . Case study on earthquake precursory phenomena , Zisin , 45 , 71 – 82 (in Japanese).
Zhang G. Zhang Z. 1992 . The study of multidisciplinary earthquake prediction in China , J. Earthq. Pred. , 1 , 71 – 85 .
Zhu F.-M. We G. 1983 . Prediction of the Haicheng earthquake and measures taken for disaster prevention , in Proc. Seminar on Earthquake Prediction Case Histories (Geneva, 1215 October 1982), pp. 1 – 18 , convened by Office of U.N. Disaster Relief Co-ordinator and UNESCO , UNDRO/83/33, GE.83-03321.
Email alerts
Astrophysics data system, citing articles via.
- Recommend to your Library
- Advertising and Corporate Services
- Journals Career Network
Affiliations
- Online ISSN 1365-246X
- Print ISSN 0956-540X
- Copyright © 2024 The Royal Astronomical Society
- About Oxford Academic
- Publish journals with us
- University press partners
- What we publish
- New features
- Open access
- Institutional account management
- Rights and permissions
- Get help with access
- Accessibility
- Advertising
- Media enquiries
- Oxford University Press
- Oxford Languages
- University of Oxford
Oxford University Press is a department of the University of Oxford. It furthers the University's objective of excellence in research, scholarship, and education by publishing worldwide
- Copyright © 2024 Oxford University Press
- Cookie settings
- Cookie policy
- Privacy policy
- Legal notice
This Feature Is Available To Subscribers Only
Sign In or Create an Account
This PDF is available to Subscribers Only
For full access to this pdf, sign in to an existing account, or purchase an annual subscription.

- News Releases
Researchers show that slow-moving earthquakes are controlled by rock permeability
University of Texas at Austin
Jackson School Associate Professor Nicola Tisato and Research Professor Harm Van Avendonk stop for a selfie in New Zealand in 2022.
Credit: Nicola Tisato / Jackson School of Geosciences
Earthquakes are the most dramatic and noteworthy results of tectonic plate movement. They are often destructive and deadly, or at the very least physically felt — they’re literally groundbreaking geological events. However not all tectonic movement results in effects that humans can perceive.
Slow slip events occur when pent up tectonic forces are released over the course of a few days or months, like an earthquake unfolding in slow motion. The more gradual movement means people won’t feel the earth shaking beneath their feet and buildings won’t collapse. But the lack of destruction does not make slow slip events less scientifically important. In fact, their role in the earthquake cycle may help lead to a better model to predict when earthquakes happen.
In a paper published recently in Geophysical Research Letters , a Jackson School of Geosciences research group led by Harm Van Avendonk, Nathan Bangs and Nicola Tisato explores how the makeup of rocks, specifically their permeability — or how easily fluids can flow through them — affects the frequency and intensity of slow slip events.
In 2019 and 2022, the group traveled to New Zealand’s North Island to collect rocks from several outcrops near the Hikurangi Margin. This is a subduction zone off New Zealand’s coast where slow slip events occur routinely, about once a year. The researchers brought back a cache of rocks to UT, where they tested their permeability and elastic properties.
Their tests showed how pores in the rocks could control the regular slow slip events at this subduction zone. Previous studies have suggested that a layer of impermeable rock at the top of the descending tectonic plate serves as a sealed lid, trapping fluid in the pores of underlying rock layers. As fluid accumulates beneath the seal, the pressure builds, eventually becoming high enough to trigger a slow slip event or earthquake. This event then breaks the impermeable seal, temporarily fracturing the rocks, allowing them to soak up fluids. Within a few months, the rocks heal and return to their initial permeability, and the cycle starts all over again.
In studying this cycle, Tisato and other researchers tested rocks from nearby surface outcrops which were once part of the earthquake fault deep underground. Previous permeability studies have been performed only on loose sediments that have been consolidated into solid rock.
“We are showing for the first time, using rocks that are representative of those at depth, that permeability is controlling (slow slip events),” he said.
Laura Wallace, a researcher at the University of Texas Institute for Geophysics and GEOMAR in Germany, has been studying slow slip events for more than 20 years, and was the first person to record slow slip events occurring in the Hikurangi Margin. She said that this paper adds more data points to inform the time scales over which the fault zone permeability changes can take place, possibly influencing the observed slow slip event cycles.
“It adds some additional data constraints on how this fault-valve process might work, how fluid cycling could work at the subduction zone — if that’s indeed what’s driving the cyclicity of these things,” Wallace said.
The ultimate goal of this research, Tisato said, is to understand why earthquakes happen and to eventually build a convincing model that can even predict them, a code scientists have yet to crack.
He and graduate student Jacob Allen are currently analyzing rock samples from the center of the margin and testing for differences in permeability. The rocks at the northern end of this subduction zone are richer in clays than those at the southern end. Because clays are malleable and can accommodate a lot of water and other fluids, they are ideally suited to trap, fracture and channel those fluids. That could explain why slow slip events on the northern end of the subduction zone happen frequently, whereas they occur rarely on the southern end, Tisato said.
“We have to go through the exercise of understanding why in the north of the Hikurangi Margin there are slow slips, and why in the south of the Hikurangi Margin we have fewer slow slips,” Tisato said. “Because if we understand that, then we have an additional step to go towards the prediction.”
Three graduate students from the Jackson School of Geosciences also contributed to this paper: Carolyn Bland, Kelly Olsen, and Andrew Gase.
Geophysical Research Letters
10.1029/2023GL103696
Method of Research
Experimental study
Subject of Research
Not applicable
Article Title
Permeability and Elastic Properties of Rocks From the Northern Hikurangi Margin: Implications for Slow-Slip Events
Article Publication Date
23-Jan-2024
Disclaimer: AAAS and EurekAlert! are not responsible for the accuracy of news releases posted to EurekAlert! by contributing institutions or for the use of any information through the EurekAlert system.
Additional Multimedia
Do earthquake hazard maps predict higher shaking than actually occurred? Research finds discrepancy
A new study by Northwestern University researchers and coworkers explains a puzzling problem with maps of future earthquake shaking used to design earthquake-resistant buildings. The research was published May 1 in the journal Science Advances in a paper titled "Why do seismic hazard maps worldwide appear to overpredict historical intensity observations?"
Although seismologists have been making these maps for about 50 years, they know very little about how well they actually forecast shaking, because large damaging earthquakes are infrequent in any area.
To learn more, the Northwestern research team compiled shaking data from past earthquakes. These include CHIMP (California Historical Intensity Mapping Project) which combines data from seismometers with historical data (termed seismic intensity) that measures ground shaking caused by earthquakes from how it affected man-made structures and objects within the quake area. Intensity information can be gleaned from photographs of damage, first-hand or newspaper accounts, and oral history.
"We found a puzzling problem," said geophysicist Leah Salditch, the study's lead author and a recent Northwestern Ph.D. graduate. "Hazard maps for California as well as Japan, Italy, Nepal and France all seemed to overpredict the historically observed earthquake shaking intensities. The hazard maps were made by groups in different countries, but they all predicted higher shaking than observed."
In analyzing the possible causes, the research team discovered the issue was with the conversion equations used in comparing hazard maps predicting future earthquakes with actual shaking data, rather than systemic problems with the hazard modeling itself.
Salditch, who was in the research group of co-author Seth Stein, William Deering Professor Emeritus of Earth and Planetary Sciences at Northwestern, is now a geoscience peril advisor at Guy Carpenter & Company. Other Northwestern authors are Molly Gallahue and James Neely, also recent Ph.D. graduates from Stein's group.
Seismologists often say that "earthquakes don't kill people, buildings kill people"—most deaths in an earthquake are caused by collapsing buildings. As a result, society's best way to reduce deaths in future earthquakes is to construct buildings that should withstand them. However, because earthquake-resistant construction is expensive, communities need to balance its costs with other societal needs. For example, they can decide to put more steel in school buildings or hire more teachers.
To make these tough choices and design appropriately, policymakers and engineers use earthquake hazard maps that predict how much shaking to expect with certain probability over the many years buildings and other structures will be in use. These maps are based on assumptions about where and how often earthquakes in the area may happen, how big they will be and how much shaking they will cause.
In delving into the puzzle of why hazard maps from five different countries all predicted higher shaking than observed, the research team figured there had to be a problem with the maps, the data or both.
"We looked at a number of possible problems with the maps, including the extent that ground shaking depends on local geology, but none of these were big enough to explain the problem," Gallahue said.
If the problem wasn't in the maps, was it in the historical data?
"Probably not," said co-author Susan Hough from the U.S. Geological Survey. "The shaking data in the different countries were compiled using different techniques but were all lower than the maps predicted. If anything, historical intensities are expected to be inflated because historical sources tend to emphasize the most dramatic effects of shaking."
If there were no problems with the hazard maps and shaking data, why didn't they agree?
"There's a subtle problem," said co-author Norman Abrahamson of the University of California, Berkeley. "Hazard maps are quoted in physical units, whereas intensities are measured on a different scale, so one must be converted to the other. It turns out the conversion equations don't work that well for very strong shaking, so converting the map values overpredicts the intensity data.
"The problem isn't the maps but in the conversion," he said. "Changing the conversion solves most of the misfit between the maps and data. Moreover, a better description of the ground shaking should make things even stronger."
"This is an important and satisfying result," said co-author Neely, now at the University of Chicago. "Maps and data that seemed not to agree were both right. The problem was in comparing the two."
"We started this project 10 years ago and thought there might be serious problems with the hazard maps," Stein said. "Now it looks like there's no fundamental problem with them.
"Maps for some areas may not be that good for various reasons," he said.
"For example, in some places we don't know enough about the earthquake history or the shaking that large earthquakes produce because of the relatively short time span available. In others, the rate and size of earthquakes may be changing or just poorly understood. So, in some places, maps may overpredict future shaking and in others they may underpredict.
"Nature will sometimes surprise us. However, because the basic hazard mapping method looks sound, we can expect these maps to be fairly good and get better as we learn more."
More information: Leah Salditch, Why do seismic hazard maps worldwide appear to overpredict historical intensity observations?, Science Advances (2024). DOI: 10.1126/sciadv.adj9291 . www.science.org/doi/10.1126/sciadv.adj9291
Provided by Northwestern University
Utilizing digital technologies for rapid damage assessment and reconnaissance: the February 6, 2023 Kahramanmaraş-Türkiye earthquakes (Mw 7.7 and Mw 7.6)
- Original article
- Open access
- Published: 09 May 2024
Cite this article
You have full access to this open access article
- Ahmet Bahaddin Ersoz 1 ,
- Onur Pekcan ORCID: orcid.org/0000-0003-3603-5929 1 ,
- Murat Altun 2 ,
- Turker Teke 2 &
- Ozgur Aydogmus 2
This paper presents a comprehensive overview of the rapid damage assessment and reconnaissance efforts following the devastating earthquakes on February 6, 2023, in Türkiye. It specifically focuses on implementing the SiteEye Disaster Plugin, an additional component of SiteEye software developed by i4 Company engineers and Middle East Technical University researchers. This tool played a critical role in managing and analyzing a massive dataset comprising over 28,000 images and videos. The research highlights the plugin’s innovative features, such as offline data collection, georeferenced-based layering, and an integrated damage classification system, significantly improving earthquake impact assessments’ accuracy and efficiency. It also underscores the importance of interdisciplinary collaboration involving national and international teams and the role of open data in disaster management. The findings demonstrate how digital technologies can transform the field of disaster response, offering new approaches for rapid assessment and effective management in the aftermath of seismic events. This research contributes valuable insights into enhancing disaster preparedness and response strategies, particularly in earthquake-prone areas.
Avoid common mistakes on your manuscript.
1 Introduction
On February 6, 2023, a sequence of devastating earthquakes struck Türkiye, leaving an unforgettable mark on history. These seismic events along the East Anatolian Fault (EAF), a zone of significant seismic activity, had far-reaching consequences for the southern and eastern parts of the nation. The initial quake’s moment magnitude (Mw) was 7.7, near Pazarcık, Kahramanmaraş, at 04:17 local Türkiye time (UTC + 3). Subsequently, a 7.6 earthquake occurred near Elbistan in Kahramanmaraş at 13:24 local Türkiye time (UTC + 3) (AFAD 2023 ). These seismic events occurred at depths of 8.6 km and 7.0 km, respectively, and were profoundly perceived over an extensive surface area. The first earthquake resulted in a fault approximately 190 km in length and 25 km in width. The subsequent earthquake occurred roughly 90 km to the northeast of the initial event. This second seismic event produced a rupture around 120 km long and 18 km wide (USGS 2023 ).
The earthquakes had geographical and social consequences in Türkiye, affecting more than 15 million people across eleven cities and an area of nearly 100,000 km 2 . Additionally, the earthquakes Mw 7.7 and Mw 7.6 shook portions of northern Syria. More than 35,000 structures collapsed, and nearly 180,000 were severely damaged due to the shaking, with most of the damage occurring in Hatay and Kahramanmaraş (TMMOB 2023 ). The casualty toll stood at around 50,000 fatalities and 107,000 injuries, according to the Ministry of Interior of Türkiye, highlighting the tragic human impact of the disaster (TRT Haber 2023 ). The widespread destruction caused by this event had a profound impact on numerous infrastructures—including industrial and residential structures, bridges, and transportation networks—resulting in economic losses surpassing $100 billion, with industrial facility damage accounting for a considerable portion of these losses (Avcil et al. 2023 ; Ozkula et al. 2023 ; Wang et al. 2023 ). Many reinforced concrete buildings in the earthquake region were severely damaged or collapsed entirely (Avcil 2023 ; Binici et al. 2023 ; Mertol et al. 2023 ; Vuran et al. 2024 ). In addition, cultural heritage sites, such as mosques and churches, also underwent damage and collapsed at multiple levels (Boyoğlu et al. 2023 ; Işık et al. 2023 ; Onat et al. 2023 ).
The earthquake’s devastation implied the criticality of efficient emergency response and disaster management procedures where digital information is vital. It was suggested that data collection and analysis efficiency in disaster reconnaissance might be improved using digital data modeling and communication strategies for information transfer (Schroeder et al. 2016 ). Earthquake databases, which contain a significant volume of images collected by engineering teams following hazard events, highlight a growing trend toward leveraging digital data sources for earthquake reconnaissance (Choi et al. 2022 ). Furthermore, increasing earthquake reconnaissance tasks utilize crowdsourcing and social media platforms as data sources, reflecting the trend toward combining digital data sources in earthquake reconnaissance (Contreras et al. 2021 ). The Preliminary Virtual Reconnaissance Report (PVRR) on the M7.2 Nippes Earthquake in Haiti (Kijewski-Correa et al. 2021a ) underscores the significance of digital platforms in enhancing the speed and accuracy of damage assessments. The “Did You Feel It?” (DYFI) system by Wald et al. ( 2011 ) exemplifies leveraging internet-based tools for rapid, widespread public engagement in seismic data collection. The integration of the MyShake smartphone application for crowdsourcing earthquake reports, as discussed by Kong et al. ( 2023 ), underscores the increasing dependence on digital technology for collecting seismic data.
In addition to underlining the utility of several earthquake missions, the significance of digital data collection utilizing omnidirectional images during post-earthquake reconnaissance missions has been underscored (Rossetto et al. 2014 ). Moreover, using unmanned aerial vehicles (UAVs) for aerial observation and rapid damage assessment in disaster management has been acknowledged as a crucial instrument for collecting digital data after seismic events (Stone et al. 2018 ). Likewise, reconnaissance organizations that collect wind, seismic, and coastal damage data during field reconnaissance missions upload and publish this information, emphasizing the significance of digital data sharing and dissemination in disaster risk management (Zwegliński 2020 ).
The comprehensive study on the catastrophic consequences of the Türkiye earthquake sequence highlights the need for digital innovation (Aktaş et al. 2023 ). The Structural Extreme Events Reconnaissance (StEER) Network’s community-centered approach (Kijewski-Correa et al. 2021b ) illustrates the role of digital tools in facilitating rapid knowledge sharing and data collection. The examination of traditional buildings’ seismic performance (Aktas et al. 2022a ) and the hybrid reconnaissance mission methodologies (Aktas et al. 2022b ) further reflect on the utility of digital and remote sensing methods in assessing earthquake impacts. The utilization of remote post-earthquake reconnaissance methodologies in response to the Haiti earthquake (Whitworth et al. 2022 ) and the evolving landscape of disaster reconnaissance missions (Aktas and So 2022 ) underscore the shift towards digital and hybrid approaches in disaster management.
The literature study illustrates the growing dependence on enhanced data modeling, remote sensing methods, and digital data for earthquake reconnaissance and disaster management (Giardina et al. 2023 ; Rathje and Franke 2016 ; Zwegliński 2020 ). Several tools have been developed that leverage digital data for post-disaster damage assessment and recovery. Roeslin et al. ( 2018 ) introduced a comprehensive tool for assessing building damage after earthquakes, effectively utilized in Mexico City following the 2017 Puebla earthquake. This tool, aiming for global applicability, includes detailed sections for recording various aspects of building damage and characteristics, underscoring the necessity for uniform methodologies in damage assessment. Similarly, Behrouzi and Pantoja ( 2018 ) focused on creating a software tool that analyzes high-resolution image data for structural damage post-earthquakes. Their tool simplifies the image tagging process, enhancing the training of deep learning algorithms for automatic damage identification. Additionally, Lin et al. ( 2019 ) developed the Real-time Individual Asset Attribute Collection Tool (RiACT), a framework for capturing attribute data of assets before and after disasters. RiACT facilitates efficient data capture, real-time transfer, and access to historical asset information, thereby supporting improved decision-making in disaster response. These tools represent significant advancements in integrating digital disaster management and recovery technology.
In light of these, the present study investigates the significance of data gathering and digital data management following the Kahramanmaraş-Türkiye earthquakes. It emphasizes the criticality of efficient data management practices for researchers to collect, analyze, and interpret enormous volumes of information to rapidly understand the scale of damage, identify weaknesses, and assess the performance of structures. It also implied the proper use of knowledge acquired from these analyses in guiding policymakers, revising design codes, and improving subsequent evaluations of earthquake risk.
During the 6th February Earthquakes, effective planning of large-scale, coordinated reconnaissance operations was possible by integrating diverse digital technologies, which also permitted instant access to field-acquired data (Cetin and Ilgac 2023 ). Researchers from several academic fields visited the affected locations, utilizing their respective areas of knowledge to analyze and record the effects on the infrastructure. Figure 1 displays images collected through the SiteEye Disaster Plugin from various locations, including reinforced concrete buildings, roads, and coastal structures, illustrating the diversity of both aerial and ground-level perspectives users provide. The collection of data was facilitated by national teams of professors from diverse universities, the Disaster and Emergency Management Presidency (AFAD), and the Ministry of Environment, Urbanization and Climate Change. Furthermore, the data-gathering procedure was joined by international teams such as EEFIT (Earthquake Engineering Field Investigation Team), GEER (Geotechnical Extreme Events Reconnaissance), and CAEES (The Canadian Association for Earthquake Engineering and Seismology). The excellence and scope of the gathered data are enhanced by this interdisciplinary collaboration, which takes place on a national and global scale; consequently, a more integrated knowledge of the effects of earthquakes is developed.
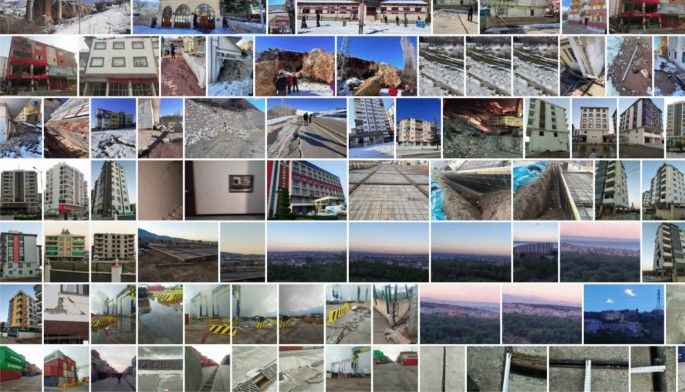
Data collected by researchers
The utilization of SiteEye ( www.siteeye.co ), a visual data management and cloud-based photogrammetry software, represents significant progress in digital data management. To respond to the earthquakes that struck Türkiye, engineers from i4 Company and researchers from the Department of Civil Engineering and Middle East Technical University worked together to develop the SiteEye Disaster Plugin voluntarily. This plugin is designed to facilitate earthquake examinations, enabling the integration of geolocated site data, including videos, drone footage, and ground images. The system handles the information effectively, merges the data with earthquake map layers, and facilitates damage interpretation. The data gathered consists of over 80 videos and more than 28,000 images, of which over 5,600 have labeled damage types immediately available to the users.
While SiteEye and tools like Fulcrum ( www.fulcrumapp.com ) and Survey123 ( www.survey123.arcgis.com ) aim to facilitate post-earthquake assessments, they differ significantly in customization and data integration capabilities. Fulcrum and Survey123 represent field data collection tools that allow users to customize and tailor forms to meet their specific requirements. In contrast, the SiteEye Disaster Plugin does not allow users to modify damage assessment forms; however, it has been methodically refined by incorporating expert feedback. Beyond the collection of damage classification data, as previously outlined, SiteEye enables researchers to upload either 2D/3D models, including orthophotos/point clouds, or generate such models through photogrammetry employing UAV photographs, thereby facilitating comprehensive terrain analysis. Furthermore, SiteEye integrates various seismological data sets, such as fault lines, surface ruptures, moment magnitude (Mw), peak ground acceleration (PGA), and peak ground velocity (PGV) distributions, into interactive maps as supplementary information layers, thereby presenting a multifaceted perspective on the impact of earthquakes.
Reflecting on the utility of the SiteEye Disaster Plugin, it is evident that its development was timely and critical for enhancing the effectiveness of earthquake reconnaissance efforts. This tool was designed and developed shortly after the two catastrophic earthquakes. It comprises a collection of purposefully engineered functionalities to optimize and enhance damage assessment investigations. The researchers’ improved efficiency and accuracy in data collection and evaluation, made possible by the SiteEye Disaster Plugin, exemplifies the platform’s critical contribution to disaster management. The procedures for data collection, management, and analysis utilizing the SiteEye Disaster Plugin are elaborated upon in the following sections of this paper. This demonstrates how digital technologies transform field studies conducted after earthquakes and provide novel approaches to rapid damage assessment and reconnaissance.
2 Development of the plugin
SiteEye, a cloud-based data management system, was created by developers and researchers affiliated with Middle East Technical University. Featuring a multi-level architecture, it efficiently facilitates data storage in the cloud and operates on several platforms, including web, iOS, and Android. SiteEye is utilized for construction site documentation, enabling extensive data collection through web and mobile applications. The mobile application efficiently manages ground data, gathering media directly from construction job sites to support detailed monitoring and reporting. Figure 2 illustrates the user interfaces of the SiteEye mobile application, exemplifying its application in collecting earthquake imagery data. SiteEye is distinguished by its georeferenced-based layering technology. This approach facilitates the utilization of diverse geographical layers, which serve as a data collection and processing framework. The software’s strength lies in its capability to autonomously extract geographic coordinates from the EXIF and embedded metadata of files, therefore cartographically representing this information with pinpoint accuracy.
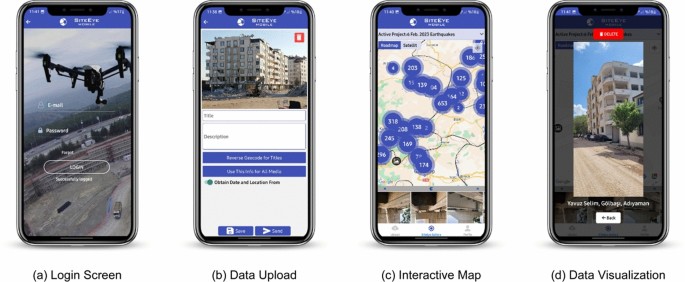
Mobile platform interfaces of SiteEye Disaster lugin
In the aftermath of two earthquakes, authors have explored strategies to enhance support for researchers conducting field investigations. Within the initial week, researchers initiated the collection of ground images from various affected cities, with damage classification labels determined through consultation with earthquake experts. After the beginning of SiteEye Disaster Plugin development, deficiencies in SiteEye’s web and mobile platform were identified by both the development team and researchers. One of the first challenges was rendering media annotations within the map and list as the dataset expanded. Performance optimizations were instituted to mitigate this, including adopting virtual scrolling for the list and clustering annotation markers on the map based on zoom level. Additionally, absent functionalities on the mobile application, such as map-view and saving media for later upload under optimal service conditions, were addressed.
Managing substantial quantities of data is an essential component of SiteEye’s operation. SiteEye platform has been used in the field surveying industry for the last seven years, handling the visualization of gigabytes of mesh and point cloud data generated using UAV (Unmanned Aerial Vehicle) imagery on a web browser, which makes it suitable for handling the substantial quantities of image data an earthquake aftermath provides. In the month following the February 6, 2023 earthquakes, 28,000 images and videos were uploaded to the servers, and it was critical to preserve optimal system performance. In order to handle this, the system implements clustering methods for proper visualization. By utilizing marker clustering, data locations are displayed on-screen at many zoom levels (Fig. 3 ). In this figure, markers are colored red if the corresponding cluster contains more than 15 images, blue if it contains fewer, and they are green if it is a singular image. This attribute is particularly critical as it facilitates the organization of enormous quantities of data into feasible segments, categorized based on the contributions of the researchers. Enriching the dataset with openly available geotagged data and facilitating data uploads from affected citizens was initially considered for data collection. However, given the plugin’s principal aim of aiding earthquake researchers in their investigations, it was decided to restrict data collection and labeling exclusively to these experts. Despite data collection and processing being undertaken by these experts, the decision to share this data for broader research endeavors was made and implemented by collecting access requests from individual researchers and groups.
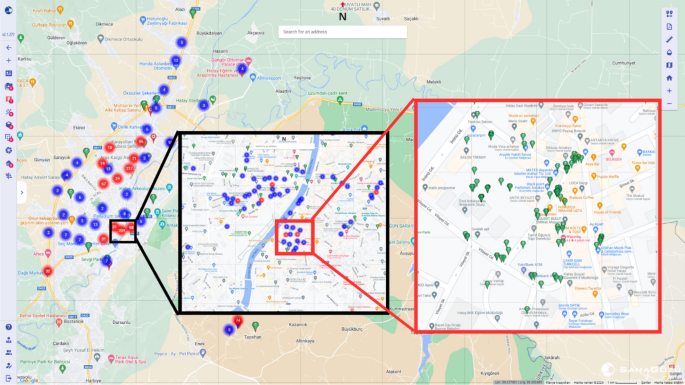
Marker clustering on the web interface
Due to the extensive disruptions in communication and internet access during the earthquakes, SiteEye modified its data collection methodologies to accommodate the challenging site conditions. Researchers can collect data in various ways using the mobile application: when internet access is available, they can shoot photographs or videos with direct geolocation tagging and upload them to SiteEye; otherwise, they can gather data offline for subsequent upload. When internet connectivity is restored, geolocation information data can be loaded into the application from the mobile device’s gallery.
SiteEye Disaster Plugin has been thoughtfully constructed to assist researchers in accurately and effortlessly classifying and evaluating data. An essential component of this framework is integrating a damage classification system. The interface incorporates this system, which enables field researchers to efficiently categorize their data according to the observed degree of damage and the type of structure. Determining the level of earthquake damage and strategizing for subsequent recovery and reconstruction endeavors require this classification. An exhaustive effort was devoted to defining classes throughout the construction of this classification system, which included consultation with earthquake experts from various fields. The classes of damages are given in Table 1 in the Appendices. The building damage categories are based on the official classification of earthquake damages on the structures reported by the Ministry of Environment, Urbanization and Climate Change, Republic of Türkiye ( 2024 ), given in Table 2 in Appendices. Immediately classified damage data benefited the engineers and researchers working in the field for reconnaissance studies as the damage was distributed in 11 provinces. This way SiteEye Disaster Plugin contributed to proper guidance for the reconnaissance. An illustrative example of the SiteEye Disaster Plugin’s impact on ground operations involves the route selection of international teams. Before their field visit, they checked the SiteEye platform to select areas they intended to focus on more closely. Specifically, the GEER team actively utilized the SiteEye platform for their visiting area selection, significantly optimizing their reconnaissance efficiency. Moreover, they also contributed their data to the platform, enriching the database with firsthand, valuable insights from the field. This synergy between SiteEye and its users facilitated targeted reconnaissance efforts and fostered a collaborative environment for data sharing and analysis.
Furthermore, the web interface, illustrated in Fig. 4 , has undergone optimization to simplify and enhance the user experience while selecting damage types, contributing to overall data analysis and reporting efficiency. Specifically, SiteEye introduces a feature allowing users to create title and description records for each photo beyond damage classifications. This capability enables users to input detailed text descriptions and pertinent tags for every image, making the dataset more informative and searchable. For instance, a user might tag a photo with “collapsed bridge or main street,” providing specific context that can be filtered and queried. This attribute is particularly advantageous for researchers, allowing them to explain their field data comprehensively. Such detailed records prove beneficial, especially when the collected data is queried by institutions and researchers from countries outside Türkiye, allowing for precise filtering based on text descriptions.
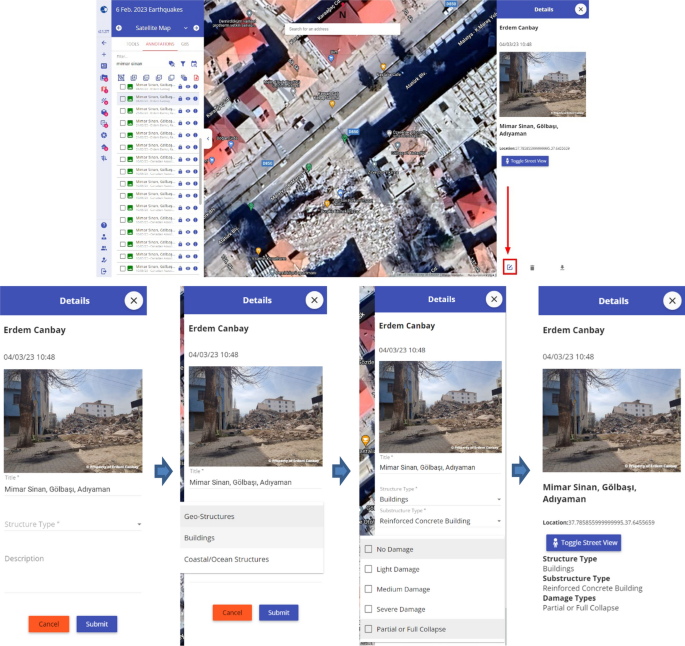
Damage classification steps
A disclosure method was implemented to attribute researchers’ contributions regarding intellectual property and data ownership. The researcher’s name obtaining the data is watermarked on every image published to the portal. In a collaborative context, this function is critical for preserving the integrity of the data and acknowledging the efforts of individual researchers.
One notable improvement to SiteEye technology is the use of Google Street View imagery. Google Street View has become a highly beneficial instrument in earthquake damage assessment, performing virtual inspections of constructed surroundings. Evangelista et al. ( 2022 ) emphasized Google Street View’s capability to capture vital information required to assess the effects of disasters on urban infrastructure. Thus, the inclusion of this functionality emerged from the requirement of researchers to observe the state of the built environment and its components before the earthquake to facilitate comparative analyses (Fig. 5 ). Employing this integration, researchers are granted access to Google Street View images that precisely align with the geographical coordinates of the data they provide.
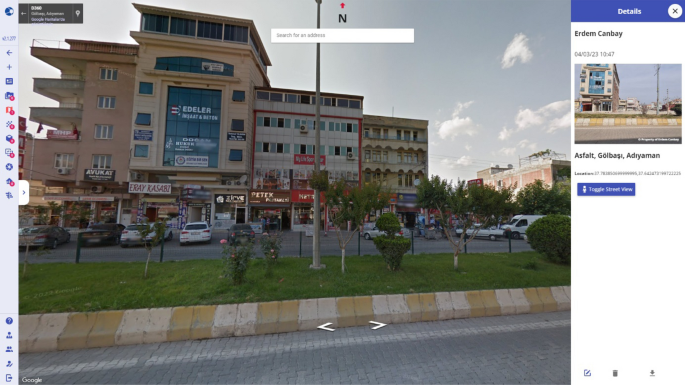
Street view comparison
3 Data collection and processing
Data acquisition for the post-earthquake assessment was initiated immediately after the earthquakes. Rapid mobilization of national and international research teams to the earthquake-affected regions followed. Data was collected with the assistance of these teams, including images and videos obtained from the ground and aerial imagery utilizing UAVs. The timely accessibility of information was pivotal in enabling the systematic organization of reconnaissance operations. A critical component of this data collection effort was the consistent continuation of communication with field researchers. This communication was crucial in informing researchers on adequately utilizing the SiteEye tool. In addition, it facilitated the prompt reporting of any discovered problems in real-time, empowering the SiteEye Disaster Plugin development team to resolve concerns immediately and guarantee the application’s seamless operation within demanding field conditions.
The primary method for collecting field data involved using the SiteEye mobile application and its corresponding web plugin. The framework of data flow is given in Fig. 6 . Following the data collection, the data processing phase contained a sequence of procedures to manage the gathered images and videos efficiently. These procedures included sorting and categorization based on geographic and structural attributes, tagging with predefined classifications, geocoding to enhance searchability and analysis, the integration of seismological data for multidimensional analysis, and utilizing the photogrammetry engine capabilities to generate detailed 3D models from aerial imagery for advanced spatial analysis. However, before delving into these processes, it was crucial to visualize the data on a map first. This initial step of visual representation highlighted the geographical distribution of the earthquake’s consequences across the impacted regions, serving as a foundational activity for subsequent analysis and decision-making processes (Fig. 7 ).
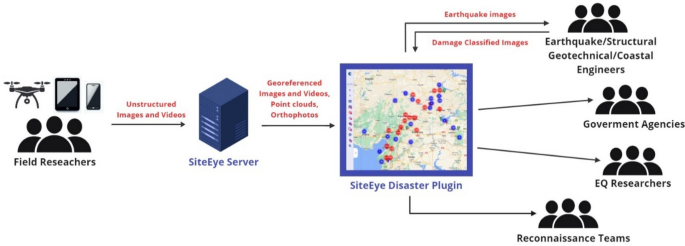
Framework of rapid earthquake reconnaissance via SiteEye Disaster Plugin
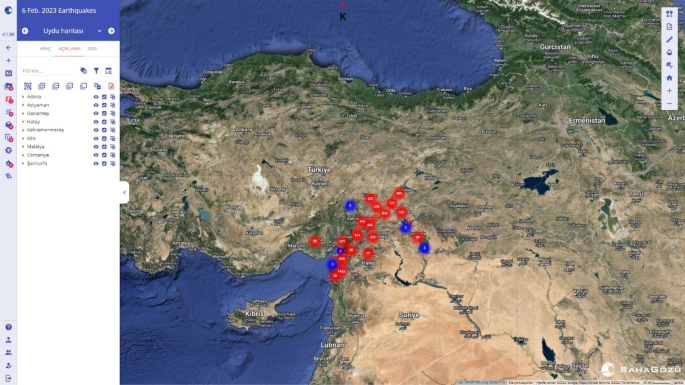
Data distribution on the map
In order to further enhance the practical interpretation of the information, the data was carefully labeled with the predetermined classifications using the structure type, substructure type, and types of damage among these particulars. Experts from numerous disciplines, including coastal, geotechnical, and structural engineering, compiled an exhaustive list from which the tags were chosen. A total of 5658 images were classified. 4387 geotechnical photos were incorporated into this comprehensive classification, which yielded vital insights regarding the earthquake’s geotechnical/geological consequences. Furthermore, 1160 images were allocated for evaluating the buildings, and 111 images were explicitly targeted at coastal structures to underscore the earthquake’s impact on these vital assets. The majority of data in SiteEye is primarily geotechnical because the platform’s user base consists mainly of geotechnical researchers, leading to a higher volume of contributions in this area. The graphical distribution of the specifics of these classes can be seen in Figs. 8 , 9 , and 10 . The values presented alongside the feature titles represent the number of photos in the figures.
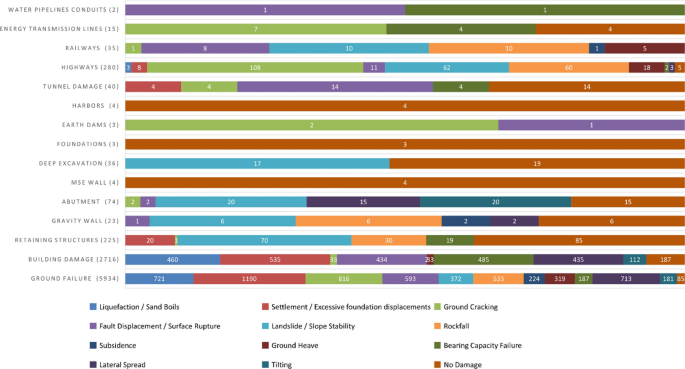
Damage classification of geotechnical structures
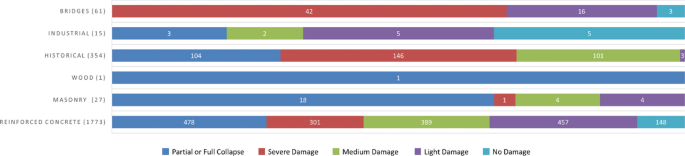
Damage classification of buildings
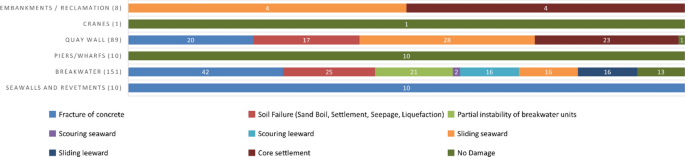
Damage classification of coastal structures
An integral component of the data processing procedure entailed geocoding the image coordinates. Geocoding involves converting geographic coordinates into more comprehensible and searchable formats, such as city, district, and street names. The incorporation of geographical information into the images significantly facilitated the process of filtering and classifying the data. An example of tagging an image would be “Yarbaşı, Pazarcık, Kahramanmaraş,” which would enable researchers to filter images according to specific locations or cities. The organization of this data played a critical role in analyzing the spatial distribution of damage and developing targeted response strategies.
The data processing phase integrated various seismological data into the mapping and imagery systems. The components mentioned above comprised fault lines, surface ruptures, moment magnitude (Mw), peak ground acceleration (PGA), and peak ground velocity (PGV) distributions. By utilizing the layering technique, it was possible to represent various data types simultaneously, thereby providing a multidimensional view of the earthquake’s effects. Those map layers are given in Fig. 11 . SiteEye Disaster Plugin, in this sense, uniquely delivered this information online as it interacted with the field researcher’s findings and provided them to others.
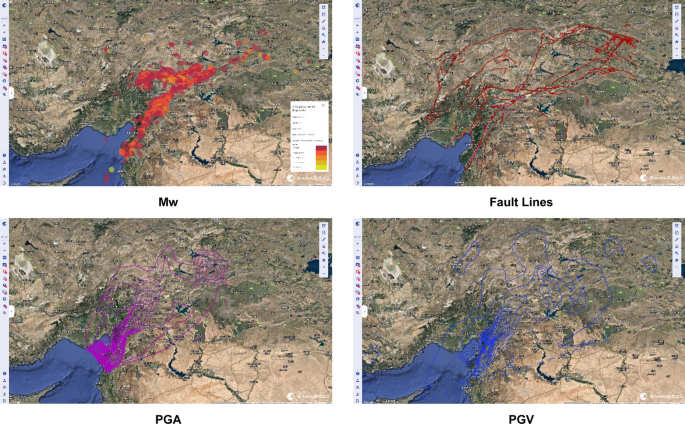
In addition to its collection and visualization of standard imagery and videos, the SiteEye software leverages photogrammetry techniques to transform aerial imagery into three-dimensional models. This process predominantly utilizes drone images, ideal for capturing high-resolution data of the earth’s surface from various angles. Through a sophisticated Structure from Motion (SfM) (Schonberger and Frahm 2016 ) and Multi-View Stereo (MVS) (Furukawa and Ponce 2010 ) pipeline, SiteEye constructs detailed 3D point clouds and orthophotos. This SfM-MVS methodology enables the accurate modeling of surface geometries, thereby facilitating a comprehensive analysis of physical terrain attributes. The generated 3D point clouds are particularly invaluable in assessing earthquake-damaged regions, allowing for an in-depth inspection of buildings with structural damage. This capability aids in the immediate evaluation of the damage and the strategic planning of reconstruction efforts. Utilizing the photogrammetry capabilities of the SiteEye software, several earthquake-affected regions were successfully transformed into detailed 3D models.
Meanwhile, the orthophotos produced by SiteEye provide an essential bird’s-eye view of the affected areas, making it possible to monitor the availability of tent areas—where tents were installed for earthquake victims—. Figure 12 exemplifies this by showcasing the tent areas to shelter earthquake victims. Also, the online accessibility of the data guarantees that professionals from various geographical locations and disciplines can readily access, collaborate on, and share it. This feature facilitates seamless teamwork across different geographies, allowing users to engage in real-time discussions, make precise model adjustments, and share insights without needing physical presence. Moreover, the cloud storage capability ensures that all modifications and annotations are saved and instantly accessible to all team members, streamlining the collaborative process and enhancing the efficiency of analyzing and planning reconstruction efforts for earthquake-affected areas.
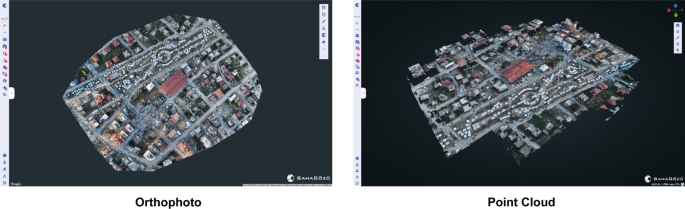
3D data generated by SiteEye
Finally, an essential field research component used markers in the mapping system to designate significant locations, such as soil sample positions. The markers functioned as critical reference points on the map, facilitating researchers and analysts to identify and examine particular sites of interest rapidly. By facilitating the correlation between physical samples and observations and the corresponding geospatial data, this function significantly enhanced the analysis and comprehension of the earthquake’s impacts as a whole.
4 Discussion
SiteEye Disaster Plugin demonstrated its ability to adapt and respond effectively to the complex data challenges from the February 6, 2023, earthquakes in Türkiye. This plugin efficiently organizes and visualizes massive datasets in order to accommodate the collection of more than 28,000 images and videos. Immediate processing and data analysis are critical for effective response and recovery action plans, making this capability crucial in disaster scenarios. This contribution emphasizes the significance of scalable and adaptable data management systems in crises, which can significantly affect the speed and efficacy of disaster relief operations.
SiteEye’s adaptable approaches to data collection effectively tackle significant obstacles in disaster management, specifically in the aftermath of such events. The software’s design incorporates protections for data collection in disaster zones, taking into account the potential disruptions in communications that may occur even in the absence of internet connectivity. This function guarantees uninterrupted data gathering, essential for the timely and detailed damage evaluation. By permitting data collection offline and subsequently uploading it once connectivity is restored, SiteEye guarantees the preservation of critical information and improves the efficiency of field operations under challenging circumstances.
The implementation of georeferenced-based layering technology in SiteEye represents a significant advancement in the visualization and management of geographical data. The capability of this technology to independently extract geographic coordinates from file metadata substantially enhances the accuracy and dependability of spatial data analysis. It facilitates the integration of diverse geographical layers, offering a more comprehensive data presentation and interpretation framework. This technological progress improves the precision of data visualization. It facilitates more informed and up-to-date decision-making in disaster response and urban planning, particularly in the aftermath of earthquakes.
SiteEye contributed substantially to disaster assessment by using photogrammetry technology to generate three-dimensional models from aerial imagery. This technology provides a dense point cloud representation of the impacted terrain, enabling in-depth topographical evaluations and spatial analyses. In understanding the extent and particulars of the damage, the capacity to produce sophisticated 3D models is essential; this capability improves the precision of assessments and facilitates the organization of reconstruction endeavors.
Developing an integrated damage classification system into SiteEye significantly advances earthquake research and disaster management. By allowing field researchers to classify data according to observed damage, this system facilitates the evaluation of earthquake impacts in a streamlined manner. The participation of experts in earthquake engineering in the process of establishing classification criteria guarantees that the system is comprehensive and pertinent. This characteristic facilitates immediate disaster response and supports critical long-term recovery planning and infrastructure resilience evaluation, substantially contributing to structural engineering and urban planning in earthquake-prone areas. This technology plays an essential role in urban planning by enabling planners to identify and map areas at high risk for earthquakes more accurately, ensuring that zoning and development regulations can be appropriately adjusted to mitigate risk. Additionally, the improved visualization technologies integral to this system aid in designing and optimizing evacuation routes, enhancing the preparedness and efficiency of emergency responses by ensuring immediate access to emergency services following a disaster.
The tool also facilitates collaborative efforts by streamlining data collection and interpretation integration between remote and on-site researchers. It aids in efficiently planning paths and strategies by utilizing previously uploaded images and analyzing the distribution of damage within specific locations. Researchers were able to plan their site visits efficiently with the assistance of classified information that was shared regarding the earthquake zone. By maximizing the coverage and efficiency of data collection efforts and guaranteeing exhaustive documentation of the extensive damage, this strategic planning was crucial. Gaining insight into the earthquake’s far-reaching effects required implementing such a methodical strategy. Additionally, its analysis was significantly impacted by the structured nature of the gathered data.
A noteworthy data analysis element was the application of Google Street View imagery captured before the earthquake. Employing SiteEye and Google Street View comparison, the destruction caused by the earthquake was clearly illustrated, offering a distinct before-and-after viewpoint. This type of imagery was crucial in understanding the extent of damage sustained by numerous structures.
The acquisition of diverse data from locations affected by widespread earthquakes is critical. It facilitates the derivation of significant patterns corresponding to the destructive nature of the occurrences. Examining and interpreting these patterns are of the highest concern for post-earthquake research, as they enhance response strategies and offer valuable insights for subsequent disaster management. For example, adapting the plugin for Istanbul, an earthquake-prone area in Türkiye, is essential to enhance earthquake preparedness. The tool’s advanced georeferenced visualizations enable emergency teams to efficiently plan and prioritize responses based on anticipated damage locations. Collaborative efforts with local stakeholders, including government agencies and educational institutions, would ensure the tool’s integration into disaster preparedness and urban planning processes. This integration enhances immediate response capabilities and fosters a community resilience and preparedness culture.
With its diverse capabilities, the SiteEye Disaster Plugin is an indispensable component of these visual inspection and analysis procedures. Some of the gathered data was made available to the public to promote collaboration and advance earthquake research. The shared dataset is an asset for researchers across the globe, facilitating a more comprehensive examination and comprehension of the consequences of the earthquake. In brief, the collective endeavors encompassing data collection, management, and analysis have substantially contributed to earthquake investigation of earthquake impacts. In addition to strengthening our comprehension of earthquake occurrences, these discoveries establish a fundamental basis for greater wisdom and efficacy in our approaches toward forthcoming earthquakes.
5 Conclusions and future works
February 6, 2023, earthquakes in Türkiye, magnitudes of 7.7 and 7.6, have underscored the critical importance of rapid and efficient disaster response and management, especially in earthquake-prone regions. The study presented here provides a comprehensive examination of the utilization of digital technologies in earthquake reconnaissance and disaster management. Integrating the SiteEye Disaster Plugin has proven to be a pivotal advancement in disaster assessment. The plugin has shown remarkable capabilities in organizing, visualizing, and managing large-scale datasets. Its features, such as offline data collection, georeferenced-based layering technology, and an integrated damage classification system, have significantly enhanced the efficiency and accuracy of earthquake impact assessments. The use of photogrammetry technology for creating detailed 3D models and incorporating Google Street View for comparative analyses further exemplifies the plugin’s contribution to understanding and managing the aftermath of seismic events. Furthermore, these data can also be utilized as educational material for training future researchers and students in earthquake engineering.
The study highlights the importance of collaborative and interdisciplinary efforts in disaster management. The involvement of national and international teams was significant in the extensive data collection and analysis process. Sharing a portion of this data as an open dataset is a testament to the commitment to collaborative research in understanding and mitigating the impacts of earthquakes. It is important to note that only a portion of the data is shared publicly due to the preferences of individual contributors, who retain the choice of whether to make their data available for open access.
In conclusion, the response to the February 2023 earthquakes in Türkiye, facilitated by the SiteEye Disaster Plugin, represents a significant leap forward in digital disaster management. The insights gained from this experience are invaluable for enhancing preparedness and response strategies for future seismic events, thereby contributing to the resilience and safety of vulnerable communities worldwide. As a future work, the integration of machine learning could help analyze and classify earthquake data, building upon the SiteEye Disaster Plugin’s capabilities. Specifically, machine learning algorithms can be trained to automatically classify images of earthquake damage, such as structural and geotechnical damage. Beyond the classification, these algorithms can also determine the severity of damage. Moreover, the algorithms can effectively compare pre- and post-earthquake images to detect damage, which is crucial for quickly identifying heavily affected areas and streamlining damage assessment. Considering these capabilities, AI-driven techniques will be developed to enhance damage assessment, perform intricate geospatial analyses, and refine photogrammetry models for more accurate 3D representations of affected areas.
Data availability
A considerable effort was initiated to reveal some of the gathered data to the public to enhance the breadth of earthquake research and promote cooperative analysis. Following this endeavor, consent was obtained from the data owners. The dataset, comprising relevant geospatial information, videos, and images, has been available to scientists and practitioners interested in earthquake investigations. In order to obtain access to this publicly available dataset, users should complete the registration process on the SiteEye platform via www.siteeye.co freely.
AFAD (2023) 06 Şubat 2023 Pazarcık (Kahramanmaraş) Mw 7.7 ve Elbistan (Kahramanmaraş) Mw 7.6 Depremlerine İlişkin Ön Değerlendirme Raporu
Aktas YD, So E (2022) Editorial: disaster reconnaissance missions: is a hybrid approach the way forward? Front Built Enviro 8:1–3. https://doi.org/10.3389/fbuil.2022.954571
Article Google Scholar
Aktaş YD, So E, Johnson C, Dönmez K, Özden AT, Vatteri AP, O’Kane A, Kalkan A, Andonov A, Verrucci E, Çabuk E, Opabola E, Malcıoğlu FS, Markov HP, Giardina G, Madabhushi G, Triantafyllou I, Byun J-E, Jones JN, Novelli V (2023) The Türkiye earthquake sequence of February 2023—A longitudinal study report by EEFIT. pp 355. https://www.istructe.org/resources/report/eefit-mission-report-turkey-february-2023/
Aktas YD, Ioannou I, Malcioglu FS, Kontoe M, Parammal Vatteri A, Baiguera M, Black J, Kosker A, Dermanis P, Esabalioglou M, Cabuk E, Donmez K, Ercolino M, Asinari M, Verrucci E, Putrino V, Durmaz B, Kazantzidou-Firtinidou D, Cotton D, D’Ayala D (2022a) Hybrid reconnaissance mission to the 30 October 2020 Aegean sea earthquake and Tsunami (Izmir, Turkey and Samos, Greece): description of data collection methods and damage. Front Built Environ 8:1–24. https://doi.org/10.3389/fbuil.2022.840192
Aktas YD, Ioannou I, Malcioglu FS, Vatteri AP, Kontoe M, Donmez K, Black J, Kazantzidou-Firtinidou D, Dermanis P, Diri-Akyildiz F (2022b) Traditional structures in Turkey and Greece in 30 October 2020 Aegean sea earthquake: field observations and empirical fragility assessment. Front Built Environ 8(May):1–17. https://doi.org/10.3389/fbuil.2022.840159
Avcil, F. (2023) Investigation of Precast Reinforced Concrete Structures during the 6 February 2023 Türkiye Earthquakes. Sustainability 15:14846. https://doi.org/10.3390/su152014846
Avcil F, Işık E, İzol R, Büyüksaraç A, Arkan E, Arslan MH, Aksoylu C, Eyisüren O, Harirchian E (2023) Effects of the February 6, 2023, Kahramanmaraş earthquake on structures in Kahramanmaraş city. In: Natural Hazards (Issue 0123456789). https://doi.org/10.1007/s11069-023-06314-1
Behrouzi A, Pantoja M (2018) Photo tagging tool for rapid and detailed post-earthquake structural damage identification. In 11th National Conference on Earthquake Engineering 2018, NCEE 2018: Integrating Science, Engineering, and Policy, 4, pp 2372–2380
Binici B, Yakut A, Kadas K, Demirel O, Akpinar U, Canbolat A, Yurtseven F, Oztaskin O, Aktas S, Canbay E (2023) Performance of RC buildings after Kahramanmaraş earthquakes: lessons toward performance based design. Earthq Eng Eng Vib 22(4):883–894. https://doi.org/10.1007/s11803-023-2206-8
Boyoğlu CS, Chike I, Caspari G, Balz T (2023) Assessing the impact of the 2023 Kahramanmaras earthquake on cultural heritage sites using high-resolution SAR Images. Heritage 6(10):6669–6690. https://doi.org/10.3390/heritage6100349
Cetin KO, Ilgac M (2023) Reconnaissance Report on February 6, 2023 Kahramanmaraş-Pazarcık (Mw=7.7) and Elbistan (Mw=7.6) Earthquakes, pp 1–484. https://doi.org/10.13140/RG.2.2.15569.61283/1
Choi J, Park JA, Dyke SJ, Yeum CM, Liu X, Lenjani A, Bilionis I (2022) Similarity learning to enable building searches in post-event image data. Computer-Aided Civil Infrastruct Eng 37(2):261–275. https://doi.org/10.1111/mice.12698
Contreras D, Wilkinson S, James P (2021) Earthquake reconnaissance data sources, a literature review. Earth (switzerland) 2(4):1006–1037. https://doi.org/10.3390/earth2040060
Evangelista MK, Aquino DH, Ramos JA (2022) Utilizing google street view for rapid seismic vulnerability assessment: case study in the city of Manila, Philippines. IOP Con Ser: Earth Environ Sci. https://doi.org/10.1088/1755-1315/1091/1/012037
Furukawa Y, Ponce J (2010) Accurate, dense, and robust multiview stereopsis. IEEE Trans Pattern Anal Mach Intell 32(8):1362–1376. https://doi.org/10.1109/TPAMI.2009.161
Giardina G, Macchiarulo V, Foroughnia F, Jones JN, Whitworth MRZ, Voelker B, Milillo P, Penney C, Adams K, Kijewski-Correa T (2023) Combining remote sensing techniques and field surveys for post-earthquake reconnaissance missions. Bull Earthq Eng. https://doi.org/10.1007/s10518-023-01716-9
Işık E, Avcil F, Arkan E, Büyüksaraç A, İzol R, Topalan M (2023) Structural damage evaluation of mosques and minarets in Adıyaman due to the 06 February 2023 Kahramanmaraş earthquakes. Eng Fail Anal. https://doi.org/10.1016/j.engfailanal.2023.107345
Kijewski-Correa T, Alhawamdeh B, Arteta C, Djima W, Do T, Mejia S, Gartner M, Gunay S, Hassan W, Javadinasab Hormozabad S, Marinković M, Martin A, Merino-Peña Y, Pájaro C, Romão X, Burlotos C, Mosalam K, Robertson I, Rodgers J, Taflanidis A (2021) Preliminary virtual reconnaissance report (PVRR). StEER: M7.2 Nippes, Haiti Preliminary Virtual Reconnaissance Report (PVRR). https://doi.org/10.17603/h7vg-5691
Kijewski-Correa T, Roueche DB, Mosalam KM, Prevatt DO, Robertson I (2021b) StEER: a community-centered approach to assessing the performance of the built environment after natural hazard events. Front Built Environ 7:1–27. https://doi.org/10.3389/fbuil.2021.636197
Kong Q, Allen RM, Allen S, Bair T, Meja A, Patel S, Strauss J, Thompson S (2023) Crowdsourcing felt reports using the MyShake smartphone app. Seismol Res Lett 94(5):2326–2336. https://doi.org/10.1785/0220230027
Lin SL, King A, Horspool N, Sadashiva V, Paulik R, Williams S (2019) Development and application of the real-time individual asset attribute collection tool. Front Built Environ 5:1–14. https://doi.org/10.3389/fbuil.2019.00015
Article CAS Google Scholar
Mertol HC, Tunç G, Akış T, Kantekin Y, Aydın IC (2023) Investigation of RC buildings after 6 February 2023, Kahramanmaraş, Türkiye Earthquakes. Buildings. https://doi.org/10.3390/buildings13071789
Onat O, Deniz F, Özmen A, Özdemir E, Sayın E (2023) Performance evaluation and damage assessment of historical Yusuf Ziya Pasha Mosque after February 6, 2023 Kahramanmaras earthquakes. Structures. https://doi.org/10.1016/j.istruc.2023.105415
Ozkula G, Dowell RK, Baser T, Lin JL, Numanoglu OA, Ilhan O, Olgun CG, Huang CW, Uludag TD (2023) Field reconnaissance and observations from the February 6, 2023, Turkey earthquake sequence. In Natural Hazards, Vol. 119, Issue 1. Springer, Netherlands. https://doi.org/10.1007/s11069-023-06143-2
Rathje EM, Franke K (2016) Remote sensing for geotechnical earthquake reconnaissance. Soil Dyn Earthq Eng 91:304–316. https://doi.org/10.1016/j.soildyn.2016.09.016
Roeslin S, Ma QTM, García HJ (2018) Damage assessment on buildings following the 19th September 2017 Puebla. Mexico Earthq Front Built Environ 4:1–18. https://doi.org/10.3389/fbuil.2018.00072
Rossetto T, D’Ayala D, Gori F, Persio R, Han J, Novelli V, Wilkinson SM, Alexander D, Hill M, Stephens S, Kontoe S, Elia G, Verrucci E, Vicini A, Shelley W, Foulser-Piggott R (2014) The value of multiple earthquake missions: the EEFIT L’Aquila earthquake experience. Bull Earthq Eng 12(1):277–305. https://doi.org/10.1007/s10518-014-9588-y
Schonberger JL, Frahm JM (2016) Structure-from-motion revisited. In Proceedings of the IEEE Computer Society Conference on Computer Vision and Pattern Recognition, 2016-Decem, pp 4104–4113. https://doi.org/10.1109/CVPR.2016.445
Schroeder GN, Steinmetz C, Pereira CE, Espindola DB (2016) Digital twin data modeling with AutomationML and a communication methodology for data exchange. IFAC-PapersOnLine 49(30):12–17. https://doi.org/10.1016/j.ifacol.2016.11.115
Stone H, Putrino V, D’Ayala D (2018) Earthquake damage data collection using omnidirectional imagery. Front Built Environ 4:1–14. https://doi.org/10.3389/fbuil.2018.00051
TMMOB (2023) Tmmob 6 Şubat Depremleri̇ 8.Ay Değerlendi̇rme Raporu. https://www.tmmob.org.tr/icerik/tmmob-6-subat-depremleri-8-ay-degerlendirme-raporu-yayimlandi . Accessed 7 April 2024
TRT Haber (2023) 6 Şubat depremlerinde 53 bin 537 canımızı yitirdik. https://www.trthaber.com/haber/gundem/6-subat-depremlerinde-53-bin-537-canimizi-yitirdik-833953.html . Accessed 7 April 2024
Türkiye Ministry of Environment Urbanization and Climate Change (2024) Damage identification. https://hasartespit.csb.gov.tr/
USGS (2023) The 2023 Kahramanmara¸s, Turkey, Earthquake sequence. https://earthquake.usgs.gov/storymap/index-turkey2023.html
Vuran E, Serhatoğlu C, Timurağaoğlu MÖ, Smyrou E (2024) Damage observations of RC buildings from 2023 Kahramanmaraş earthquake sequence and discussion on the seismic code regulations. Bull Earthq Eng. https://doi.org/10.1007/s10518-023-01843-3
Wald DJ, Quitoriano V, Worden B, Hopper M, Dewey JW (2011) USGS “Did You Feel It?” internet-based macroseismic intensity maps. Ann Geophys 54(6):688–707. https://doi.org/10.4401/ag-5354
Wang T, Chen J, Zhou Y, Wang X, Lin X, Wang X, Shang Q (2023) Preliminary investigation of building damage in Hatay under February 6, 2023 Turkey earthquakes. Earthq Eng Eng Vib 22(4):853–866. https://doi.org/10.1007/s11803-023-2201-0
Whitworth MRZ, Giardina G, Penney C, Di Sarno L, Adams K, Kijewski-Correa T, Black J, Foroughnia F, Macchiarulo V, Milillo P, Ojaghi M, Orfeo A, Pugliese F, Dönmez K, Aktas YD, Macabuag J (2022) Lessons for remote post-earthquake reconnaissance from the 14 August 2021 Haiti earthquake. Front Built Environ 8:1–16. https://doi.org/10.3389/fbuil.2022.873212
Zwegliński T (2020) The use of drones in disaster aerial needs reconnaissance and damage assessment-Three-dimensional modeling and orthophoto map study. Sustainability (Switzerland) 12(15):1–20. https://doi.org/10.3390/su12156080
Download references
Acknowledgements
We extend our sincere thanks to Furkan Aydoğan, Ediz Karaali, Yunus Eren Kaya, Görkem Kılıç, Muzaffer Ata Özbilen, and Serhat Erinmez for their exceptional support. Their expertise and commitment were crucial in advancing our project, and we are deeply grateful for their contributions. Their efforts have significantly enhanced the quality and success of our work.
Open access funding provided by the Scientific and Technological Research Council of Türkiye (TÜBİTAK). The authors declare that no funds, grants, or other support were received during the preparation of this manuscript. The aforementioned plugin was developed voluntarily.
Author information
Authors and affiliations.
Department of Civil Engineering, Middle East Technical University, Ankara, Türkiye
Ahmet Bahaddin Ersoz & Onur Pekcan
i4, Middle East Technical University Technopolis, Ankara, Türkiye
Murat Altun, Turker Teke & Ozgur Aydogmus
You can also search for this author in PubMed Google Scholar
Corresponding author
Correspondence to Onur Pekcan .
Ethics declarations
Conflict of interests.
The authors have not disclosed any competing interests.
Additional information
Publisher's note.
Springer Nature remains neutral with regard to jurisdictional claims in published maps and institutional affiliations.
See Tables 1 and 2 .
Rights and permissions
Open Access This article is licensed under a Creative Commons Attribution 4.0 International License, which permits use, sharing, adaptation, distribution and reproduction in any medium or format, as long as you give appropriate credit to the original author(s) and the source, provide a link to the Creative Commons licence, and indicate if changes were made. The images or other third party material in this article are included in the article's Creative Commons licence, unless indicated otherwise in a credit line to the material. If material is not included in the article's Creative Commons licence and your intended use is not permitted by statutory regulation or exceeds the permitted use, you will need to obtain permission directly from the copyright holder. To view a copy of this licence, visit http://creativecommons.org/licenses/by/4.0/ .
Reprints and permissions
About this article
Ersoz, A.B., Pekcan, O., Altun, M. et al. Utilizing digital technologies for rapid damage assessment and reconnaissance: the February 6, 2023 Kahramanmaraş-Türkiye earthquakes (Mw 7.7 and Mw 7.6). Bull Earthquake Eng (2024). https://doi.org/10.1007/s10518-024-01925-w
Download citation
Received : 12 January 2024
Accepted : 18 April 2024
Published : 09 May 2024
DOI : https://doi.org/10.1007/s10518-024-01925-w
Share this article
Anyone you share the following link with will be able to read this content:
Sorry, a shareable link is not currently available for this article.
Provided by the Springer Nature SharedIt content-sharing initiative
- Earthquake reconnaissance
- Digital disaster management
- Rapid damage assessment
- Collaborative research
Advertisement
- Find a journal
- Publish with us
- Track your research
- Apply to UMaine
UMaine News
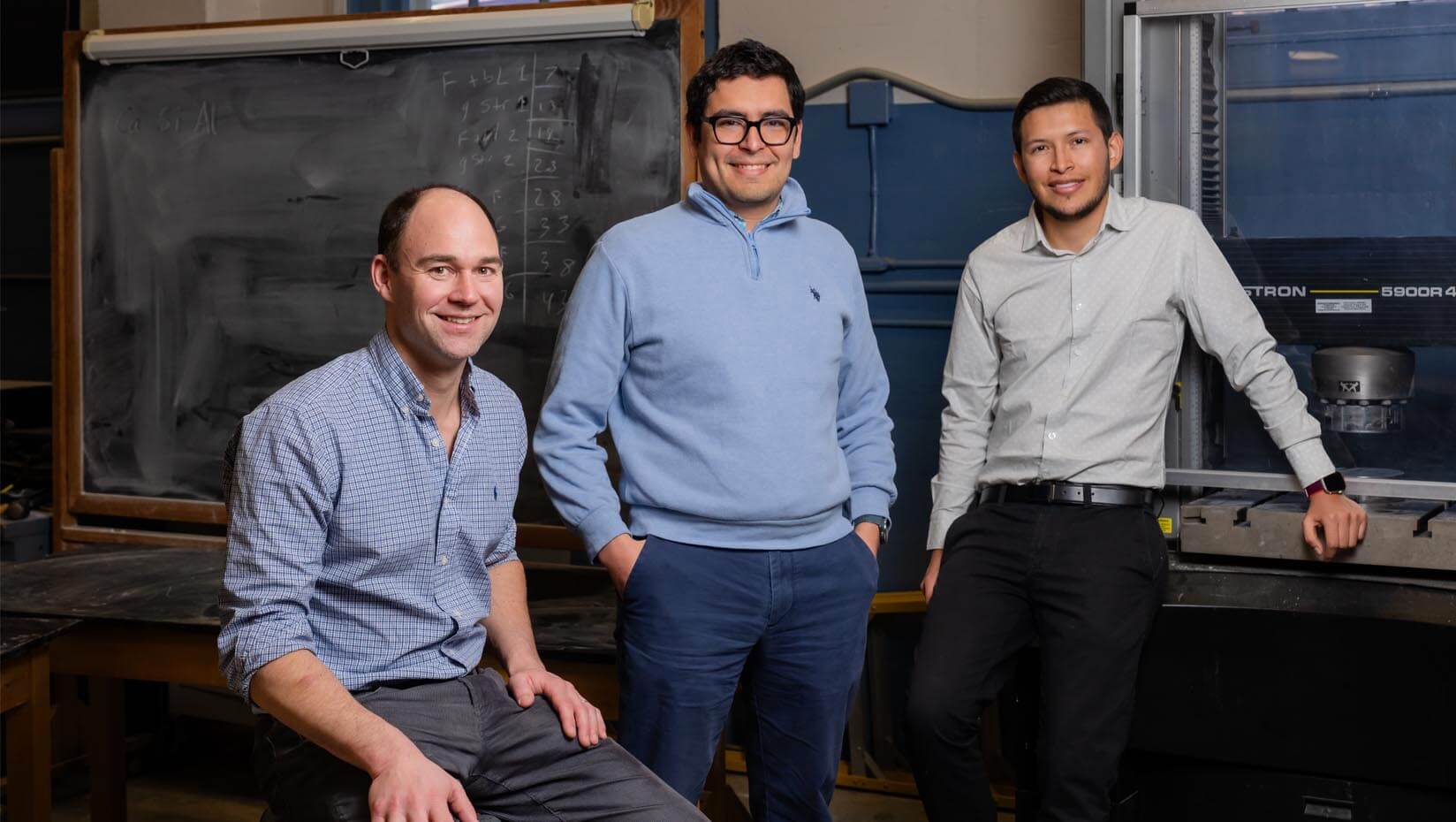
NSF backs UMaine research to lessen earthquake damage with new, inexpensive method
On the backside of a piece of paper he found at his desk, University of Maine associate professor of geotechnical engineering Aaron Gallant drew a microscopic view of soil to explain the foundation of his research. Inside a small square, he penned in circles of different sizes for two types of particles and wavy lines for groundwater flow through the soil. The smaller of the two particles represented tiny gas bubbles floating in water between soil grains, the larger of the two.
Although the soil is loose and deformable, Gallant said it’s stable as long as soil particles can compact and displace the groundwater. It can lose stability when vibrations from an earthquake create pressure in the ground and liquify soil with high water content. Known as liquefaction, the process is capable of destabilizing foundations under bridges, homes, oil tanks and other facilities, causing them to collapse.
The tiny gas bubbles represented in Gallant’s illustration may have the ability to effectively mitigate the damaging impacts of liquefaction by suppressing groundwater pressurization.
In partnership with Portland State University (PSU) in Oregon, Gallant and two other researchers from UMaine — assistant professor of civil and environmental engineering Luis Zambrano-Cruzatty and graduate student Andres Cordoba — are testing a new method of fortifying water-saturated soil. The team will be stimulating microbes in the ground that generate nitrogen gas to buffer ground pressure created by earthquakes, like shocks on a car.
The National Science Foundation (NSF) selected the team in a competitive grant process for an award of $961,871 to conduct the research, with UMaine’s share totaling $365,594.
“We’re thinking about how the ground interacts with a built environment,” said Gallant. “One of the things that is very difficult to mitigate is this idea of liquefaction, when the soil turns from a solid state essentially into what’s considered a liquid state.”
Regions prone to earthquakes and near water, such as the west coast, greater Charleston area in South Carolina and New Madrid seismic zone in the midwest, are specifically in danger of soil liquefaction in the U.S.
The inspiration
“This is something that can be very destructive,” said Gallant. “The specific site that we’re concentrating on here is the critical energy infrastructure hub in Portland, Oregon. They have a lot of oil tanks sitting on liquefiable soils. If a big earthquake happens, all of that oil and the state’s energy supplies are susceptible to this big, potentially catastrophic event.”
If the soil liquified during an earthquake, Oregon could lose 90% of its petroleum reserves .
Diane Moug, assistant professor of civil and environmental engineering at PSU, said since Oregon doesn’t have fuel refineries, all the liquid fuel is shipped to the infrastructure hub then distributed. The fuel tanks were built before the state understood the seismic hazard.
“These fuel tanks are not seismically robust,” said Moug. “They’re on soil that will fail in an earthquake.”
Besides the loss of liquid fuel for the entire state, Moug said people are concerned that if the tanks fail they will spill into the Willamette River causing harm to fisheries and ecosystems.
Since tanks and infrastructure are already there, she said improving the soil underneath is difficult. The gas-generating method the research team is studying is one of a few that can treat ground where infrastructure exists.
“There’s this nice synergy of us looking at how effective this method is for reducing earthquake hazards, and Gallant and his team are looking at how long it lasts,” said Moug. “We see those two questions as really intertwined. You can’t have one without the other.”
The multi-point research is an attempt to not only create a solution for soil liquefaction, but to make it cost effective and widely accessible — for resource-rich countries like the U.S. and other places like rural Indonesia that often experience earthquakes and are prone to liquefaction.
Following a 2018 earthquake in Indonesia, Gallant joined an NSF-funded research team associated with the Geotechnical Extreme Events Reconnaissance Association that traveled to the Southeast Asian country and studied the Palu-Donggala quake. Researchers concluded liquefaction from the earthquake had triggered catastrophic landslides that caused mass destruction and the death of more than 4,000 people.
New technique
Gallant’s research into mitigating liquefaction dates back to 2016 when he joined UMaine.
This most recent segment funded by the near $1 million NSF grant is specifically focused on fortifying silt soils over a long period of time by generating nitrogen gas with microbially induced desaturation (MID). Inexpensive compared to reinforcing building foundations or driving concrete pillars deep into the ground, the generated nitrogen gas absorbs pressure from earthquakes by filling empty space between soil particles and groundwater.
“It’d be very challenging to take a straw, blow bubbles into the soil and get it uniformly mixed,” said Gallant. “With MID, we’re essentially stimulating the microbes and getting a denitrification process rolling that will allow us to generate nitrogen gas in place.”
In addition to being a cost effective solution, Gallant said nitrogen gas isn’t damaging to the environment and will last a long time in the soil, potentially decades to a century. It doesn’t dissolve easily in water, and the atmospheric pressure of nitrogen — the gas with the highest naturally occurring concentration in the air — significantly contributes to the persistence and longevity of gas entrapped in the soil column.
“What happens when you open a Coke bottle? The CO 2 comes out,” said Gallant. “But why was it staying there before? Because it had a lot of CO 2 pressure inside the bottle.” The same concept applies to nitrogen gas in groundwater.
Two challenges will be knowing whether the gas is staying in place and predicting how long it will be there. If the research team can successfully address these concerns, MID and other desaturation techniques have the ability to transform how civil engineers protect infrastructure susceptible to liquefaction.
Contact: Ashley Yates; [email protected]
- UMaine Today Magazine
- Submit news

Popular Keywords
Research directory.
- Semiconductor Research
- Display Research
- Optoelectronics Research
- Green Energy Research
- ICT Applications Research
- Semiconductors
- Wafer Foundries
- Compound Semiconductor
- AI Server/HBM/Server
- Upstream Components
- Panel Industry
- Display Supply Chain
- Display Technologies
- LED Demand / Supply Data Base
- Sapphire Market
- LED Chip & Package
- LED Backlight
- LED Lighting
- LED Display
- IR LED / VCSEL / LiDAR Laser
- UV LED / UV Laser
- MicroLED / Mini LED
- Lithium Battery and Energy Storage
- Consumer Electronics
- Notebook Computers
- Smartphones
- Monitors / AIO
- Emerging Technologies
- Cloud / Edge Computing
- Automotive Technologies
Selected Topics
- Telecommunications
- Computer System
- Automotive & EV
- Green Energy & Storage
- Display Panel & LED
- DRAM (Index)
- TFT-LCD (Index)
- Li-Ion Battery
- Press Center
- Beta DataTrack
- [News] Controlled Market Demand Amid Earthquake Impact, Second Quarter DRAM Prices Expected to Rise by Over 20%
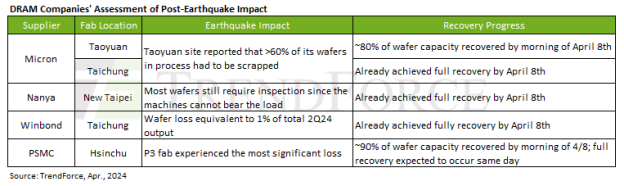
According to a report from TechNews , there has been a significant surge in demand in the memory market, driving prices steadily upward. This surge has led to the latest quotations from Korean memory manufacturers, with DDR5 prices set to increase by 13% in May.
Additionally, DDR4 prices are also expected to rise by 10%. As for DDR3, which currently serves as the main supply for Taiwanese memory manufacturers, there is also room for a 10% to 15% increase. Overall, contract prices for the second quarter are anticipated to rise by 20% to 25%.
Following in the wake of an earthquake that struck on April 3rd, TrendForce undertook an in-depth analysis of its effects on the DRAM industry , uncovering a sector that has shown remarkable resilience and faced minimal interruptions. Despite some damage and the necessity for inspections or disposal of wafers among suppliers, the facilities’ strong earthquake preparedness of the facilities has kept the overall impact to a minimum.
Leading DRAM producers, including Micron, Nanya, PSMC, and Winbond had all returned to full operational status by April 8th. In particular, Micron’s progression to cutting-edge processes—specifically the 1alpha and 1beta nm technologies—is anticipated to significantly alter the landscape of DRAM bit production. In contrast, other Taiwanese DRAM manufacturers are still working with 38 and 25nm processes, contributing less to total output. TrendForce estimates that the earthquake’s effect on DRAM production for the second quarter will be limited to a manageable 1%.
With the earthquake’s impact under control, for each manufacturer, Micron temporarily suspended quotations due to the earthquake’s impact on production. After completing the assessment of losses, it notified customers of a 25% increase in DRAM and SSD contract prices.
Additionally, due to Samsung’s production line conversion, the early cessation of DDR3 production has prompted many customers to turn to Nanya and Winbond for procurement orders, leading to the completion of product verification. As a result, Nanya and Winbond have informed customers in the second quarter that DDR3 prices are expected to increase by 10-15%.
Per TrendForce’s observations, as the three major memory manufacturers continue to transition their production capacity to highly demanded products such as HBM and DDR5, the production capacity of products like DDR4 and DDR3 has significantly decreased.
Overall, the supply-demand gap is expected to exceed 20-30% by the second half of 2024. This situation will lead to a substantial increase in DDR3 prices in the second half of the year, with price hikes reaching 50-100% as current prices remain below costs. This scenario will also be advantageous for the operational performance of various domestic memory manufacturers.
- DRAM Manufacturers Gradually Resume Production, Impact on Total Q2 DRAM Output Estimated to Be Less Than 1%, Says TrendForce
- [Insights] DRAM Spot Prices Expected to Decline Post-Manufacturer Quoting Resumption
Please note that this article cites information from TechNews .

Related Articles
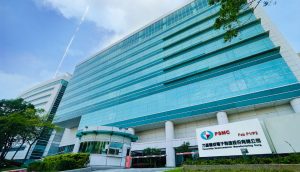
- [News] PSMC’s New Tongluo Plant Unveiled, CoWoS Packaging Ready to Roll
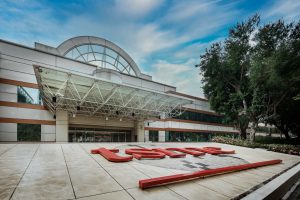
[News] TSMC Reportedly Commences Production of Tesla's Next-Generation Dojo Chips, Anticipates 40x Increase in Computing Power in 3 Years
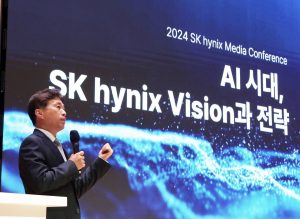
[News] HBM Craze Continues! SK Hynix Reports Sold Out for this Year, Next Year's HBM Capacity Nearly Fully Booked
- Search for:
Recent Posts
- [News] TSMC Reportedly Commences Production of Tesla’s Next-Generation Dojo Chips, Anticipates 40x Increase in Computing Power in 3 Years
- [News] HBM Craze Continues! SK Hynix Reports Sold Out for this Year, Next Year’s HBM Capacity Nearly Fully Booked
- [News] AI Chip Alone Can’t Hold Up? AMD’s Fiscal Forecast This Quarter Reportedly Falls Short of Expectations
Recent Comments
- Panel Costs Account for More Than 60% of 21.5-inch Monitor Retail Prices, with LCD Monitor Panel Prices Likely to Keep Rising on Panel Costs Account for More Than 60% of 21.5-inch Monitor Retail Prices, with LCD Monitor Panel Prices Likely to Keep Rising
- February 2024
- January 2024
- December 2023
- November 2023
- October 2023
- September 2023
- August 2023
- February 2023
- January 2023
- December 2022
- November 2022
- October 2022
- September 2022
- August 2022
- February 2022
- January 2022
- December 2021
- November 2021
- October 2021
- September 2021
- August 2021
- February 2021
- January 2021
- 5G Technologies
- Artificial Intelligence
- Broadband & Home Network
- IC Manufacturing, Package&Test
- Industry 4.0
- Micro LED / Mini LED
- Wearable Devices
- Entries RSS
- Comments RSS
- WordPress.org
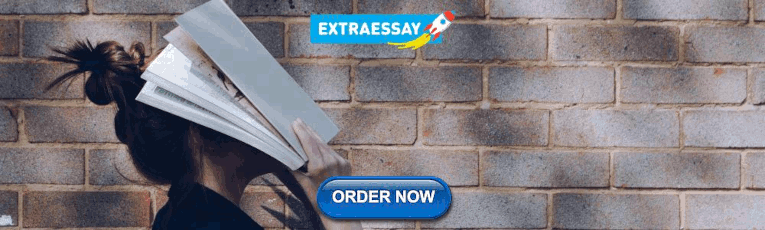
IMAGES
COMMENTS
The Coast and Geodetic Survey, a forerunner of the National Ocean Survey, had coordinated the collection of seismological data in the United States for many years. The NEIC was transferred to Boulder, Colorado, in 1972 and made part of the U.S. Geological Survey in 1973. The NEIC was moved again in 1974 to its present location in Golden, Colorado.
For comparison, the Earthquake Engineering Research Institute (EERI) (2003b) extrapolated the FEMA (2001) estimate of AEL ($4.4 billion) for residential and commercial building-related direct economic losses by a factor of 2.5 to include indirect economic losses, the social costs of death and injury, as well as direct and indirect losses to the
The aim of Earthquake Research Advances is to improve our understanding of earthquake physics, expand our ability to observe earthquake-related phenomenon and improve our mitigation of seismic hazards. To achieve that goal, the journal publishes original research articles focused on all aspects of earthquake studies. ... Rapid report of the ...
A National Research Council committee will develop a roadmap for earthquake hazard and risk reduction in the United States. The committee will frame the road map around the goals and objectives for achieving national earthquake resilience in public safety and economic security stated in the current, publically available strategic plan of the National Earthquake Hazard Reduction Program (NEHRP ...
The National Earthquake Hazards Reduction Program Reauthorization Act of 2018 includes a provision for GAO to assess the program's efforts. This report examines, among other things, NEHRP's progress in identifying gaps and strengthening resilience to earthquakes, and its activities to identify and communicate about research priorities.
Marie: My research aims to understand the physics of fluid-induced earthquakes. Anthropogenic fluid injections during hydraulic fracturing, reservoir impoundment, the injection of waste water or ...
5 Conclusions— Achieving Earthquake Resilience. The advent of NEHRP in 1977, together with its subsequent reauthorizations, added substantial resources for research in seismology, earthquake engineering, and social sciences with the goal of increasing knowledge for understanding the causes of earthquakes and reducing their impacts.
Earthquake Spectra is a peer-reviewed journal with the purpose of improving the practice of earthquake hazards mitigation, preparedness, and recovery. Established in 1984, the journal is owned by the Earthquake Engineering Research Institute (EERI) and is dedicated to providing the publication of record for the development of earthquake engineering practice, earthquake codes and regulations ...
For decades, earthquake prediction has been the focus of research using various methods and techniques. It is difficult to predict the size and location of the next earthquake after one has occurred. However, machine learning (ML)-based approaches and methods have shown promising results in earthquake prediction over the past few years. Thus, we compiled 31 studies on earthquake prediction ...
Metrics. A new generation of earthquake catalogs developed through supervised machine-learning illuminates earthquake activity with unprecedented detail. Application of unsupervised machine ...
The PEER Reports Series comprises state-of-the-art research in earthquake engineering and related fields by the more than 150 expert members of the PEER universities consortium. The current emphasis of the series is on performance-based engineering of lifelines and building structures. Full-color reports are available free of charge as a PDF ...
The Sendai Framework for Disaster Risk Reduction 2015-2030 (SFDRR) highlights the importance of scientific research, supporting the 'availability and application of science and technology to decision making' in disaster risk reduction (DRR). Science and technology can play a crucial role in the world's ability to reduce casualties, physical damage, and interruption to critical ...
Geophysicists Camilla Cattania and William Frank team up to explore the tectonics and fault mechanics behind earthquakes, and their associated hazards. Landsat 8 captured this view of the folded rock landscape of Morocco's Anti-Atlas Mountains, formed by the slow-motion collision of the African and Eurasian tectonic plates.
The USGS monitors and reports on earthquakes, assesses earthquake impacts and hazards, and conducts targeted research on the causes and effects of earthquakes. We undertake these activities as part of the larger National Earthquake Hazards Reduction Program (NEHRP), a four-agency partnership established by Congress. Search Earthquake Catalog.
A normal (dip-slip) fault is an inclined fracture where the rock mass above an inclined fault moves down (Public domain.) An earthquake is what happens when two blocks of the earth suddenly slip past one another.The surface where they slip is called the fault or fault plane.The location below the earth's surface where the earthquake starts is called the hypocenter, and the location directly ...
2017. The Pacific Earthquake Engineering Research Center (PEER) is a multi-institutional research and education center with headquarters at the University of California, Berkeley. PEER's mission is to develop, validate, and disseminate performance-based seismic design technologies for buildings and infrastructure to meet the diverse economic ...
A similar design has been adopted for seismic networks in Japan. After the 1995 Kobe earthquake, the Japanese government initiated the Headquarters for Earthquake Research Promotion project aiming to construct a national shaking map and promote understanding of the long-term possibility of earthquake occurrences (Okada et al., 2004).
Regression analysis is a statistical method. used for finding relationships between independent and de-. pendent variables. Regression analysis can be used for. prediction and forecasting. In ...
The earthquak e. resistant construction is considered to be ve ry important to mitigate their effects. This paper. presents the concise prerequisites of earthquake resistant construction and a few ...
Earthquake prediction research has been conducted for over 100 years with no obvious successes. Claims of breakthroughs have failed to withstand scrutiny. Extensive searches have failed to find reliable precursors. Theoretical work suggests that faulting is a non-linear process which is highly sensitive to unmeasurably fine details of the state ...
The National Institute of Standards and Technology (NIST)-the lead NEHRP agency-commissioned the National Research Council (NRC) to develop a roadmap for earthquake hazard and risk reduction in the United States that would be based on the goals and objectives for achieving national earthquake resilience described in the 2008 NEHRP Strategic Plan.
volcanoes. Destructive earthquakes, often resulting in tsunami, occur several times each century. The 1923 Tokyo earthquake killed over 140,000 people. More recent major quakes are the 1995 Great Hanshin earthquake and the 2011 T ōhoku earthquake, a 9. 0-magnitude quake which hit Japan on March 11, 2011, and triggered a large tsunami.
In a paper published recently in Geophysical Research Letters, a Jackson School of Geosciences research group explores how the makeup of rocks, specifically their permeability — or how easily ...
The ShakeAlert Earthquake Early Warning (EEW) system aims to issue an advance warning to residents on the West Coast of the United States seconds before the ground shaking arrives, if the expected ground shaking exceeds a certain threshold. ... This report is an update to the presentation by Schulz (1989) introducing potential users to the ...
A new study by Northwestern University researchers and coworkers explains a puzzling problem with maps of future earthquake shaking used to design earthquake-resistant buildings. The research was ...
This paper presents a comprehensive overview of the rapid damage assessment and reconnaissance efforts following the devastating earthquakes on February 6, 2023, in Türkiye. It specifically focuses on implementing the SiteEye Disaster Plugin, an additional component of SiteEye software developed by i4 Company engineers and Middle East Technical University researchers. This tool played a ...
Under a multidimensional frequent earthquake, the transverse displacement of the structure increased by about 13% on average compared with that under a bidirectional earthquake; the longitudinal increase was the largest, at about 28%. ... The research reported in this paper was supported by the National Natural Science Foundation of China ...
This paper uses the survey data of "Earthquake disaster insurance literacy of Chinese residents" to construct the earthquake disaster insurance literacy index system, and analyzes the ...
Following a 2018 earthquake in Indonesia, Gallant joined an NSF-funded research team associated with the Geotechnical Extreme Events Reconnaissance Association that traveled to the Southeast Asian country and studied the Palu-Donggala quake. Researchers concluded liquefaction from the earthquake had triggered catastrophic landslides that caused ...
According to a report from TechNews, there has been a significant surge in demand in the memory market, driving prices steadily upward.This surge has led to the latest quotations from Korean memory manufacturers, with DDR5 prices set to increase by 13% in May. Additionally, DDR4 prices are also expected to rise by 10%.