- Open access
- Published: 06 January 2021
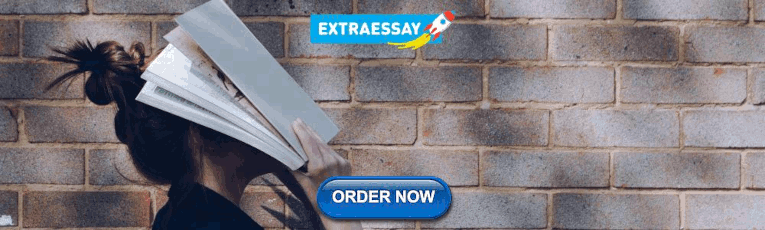
Current technology for identifying dental implants: a narrative review
- Mohammad Ali Saghiri ORCID: orcid.org/0000-0002-5064-7828 1 , 2 ,
- Peter Freag 3 ,
- Amir Fakhrzadeh 4 ,
- Ali Mohammad Saghiri 5 &
- Jessica Eid 3
Bulletin of the National Research Centre volume 45 , Article number: 7 ( 2021 ) Cite this article
5528 Accesses
17 Citations
Metrics details
This paper outlines the current status and mechanism for identifying dental implants, with emphasis on future direction and updated technology, and covers the existing factors influencing the identification of implant systems.
A search was performed on the current methods of identifying dental implants between January 2000 through Feb 2020 using online databases for articles published in English. The search was performed using the Google, Rutgers library, PubMed, MEDLINE databases via OVID using the following keywords: implant types identification by x-ray imaging, forensic identification of dental implant, surface types, threaded, non-threaded, software identification, recent technologies, which evaluated different methods in the identification of dental implants and its clinical importance for the dentist and the patient. Of the 387 articles found in initial search results, 10 met the inclusion criteria set for this review. These 10 studies were directly related to the identification of different implant systems. Many studies have indicated identifying dental implants as problematic due to many confounding factors, and the difficulty in finding the specific parts for the dental implant itself. The contribution of digital dentistry is critical. Factors like increasing number of implant manufacturers, dental tourism, and cost, make it difficult to detect and match dental implants by dentists during the chairside time.
These factors give rise to the need for a new system to help clinicians in decision making. Artificial intelligence seems to have shown potential to help in this case. However, detailed regulatory mechanisms are still needed for diagnosis and analysis.
Dental implants have become a popular choice of treatment in replacing individual lost teeth or entire dentitions. According to the American College of Prosthodontists (A, people with low income or education have fewer remaining teeth (ACP 2020 ). Also, 27.27% of seniors over age 65 have no remaining teeth (NIH 2020 ). Every year, more than 800,000 individual implants are installed in the United States (US), and more than 1.8 million in the European Union (EU) (Insights et al. 2027 ). This number is expected to increase considerably (NIH 2020 ) due to an increase in the geriatric population and the number of general dentists and specialists performing the procedure. Dental implant therapy is an invasive, lengthy, and precise procedure. Each of the components used in this process are specific to the original implant down to the manufacturer, type and size since most implant companies have a unique library of implant designs, sizes, and platforms. The amount of time it takes for an implant procedure from start to final restoration can be as long as a year in most patients and can cost upwards of $4000 per implant (site AAID. 2020 ). Since an implant contains many different components (Cappare et al. 2019 ; Takeuchi et al. 2018 ), it may be difficult to replace it without the knowledge of the implant type.
The major problem with this process is that clinicians often find patients who have had implants placed in other U.S. dental offices or from areas abroad without any records regarding the identification of the implant system (Fox News 2020 ). Due to the cost of dental implant therapy in the US, more patients are traveling overseas to have implants placed and subsequently come back to the U.S. to have the implants restored. Currently, identification of the specific implants, without patient records based on radiographic or clinical observation is difficult because of a lack of identifying markers on implants. This problem doesn’t just arise during the implant restoration process but is also a cause for concern when implant complications arise. The need for improved methods for accurate implant recognition is widely understood by clinicians and patients who have encountered these issues.
All the currently used methods for implant identification and classification are time-consuming and not very accurate. The most current and frequently used method for identifying dental implants is a website (whatimplantisthat. 2020 ) that simply provides photos of hundreds of X-rays that clinicians must search through individually to try to help in identifying their patient's implant after they input descriptive features of the implant to narrow down their search field. Lack of an established and efficient system for identifying dental implants, keeping all the different confounding factors including dental tourism, increasing number of implant manufacturers, and cost in mind, has proven to be a hurdle in systemized and timely identification of implants during the chairside time. Developing innovative methods to identify these previously placed implants based on radiographic and clinical data, will spare millions of patients and clinicians the difficult task of deciding whether to proceed with the very invasive unpredictable procedures to remove and replace unidentifiable implants, restore and rehabilitate them with mismatched components. This calls for identification and revaluation of the current technology for identifying dental implants so pave way for it. This paper, therefore, aims to, analyze the existing technology, the new upcoming technology, and its future direction, covering the existing factors influencing the identification of implant systems: manufacturing, patient, and imaging factors.
Materials and methods
In this review, the methods for identifying dental implants and their clinical importance for the dentist and the patient are assessed. The factors influencing dental implant survival rates and in extension, leading to an increased need for a centralized database for implants are also assessed. The main aspect of this review is to evaluate the methods in identifying dental implant systems worldwide and describing the limitations within these current methods.
Inclusion and exclusion criteria
The inclusion criteria were studies accepted and published in the English language between January 2000 through Feb 2020. The inclusion criteria included the scientific in-vivo, in-vitro articles, reviews, systematic reviews, case reports, and clinical trials with controlled study design. Studies were also included that had identified the dental implant systems, implant systems manufacturer identification, forensic radiographic identification, global dental implant market varsity, major implant manufactures, and factors affecting implant maintenance.
The exclusion criteria were studies that were published before January 2000 and through Feb 2020. Criteria also excluded the studies that focused on other restoration types to replace missing teeth. Also, excluded studies that mainly focused on other aspects of implant surgical techniques, impression systems, that do not affect the identification of the implant system.
Search methodology
A literature search was performed electronically using the Google, Rutgers library, PubMed, MEDLINE databases via OVID using the keywords mentioned in the PubMed and MeSH headings for articles published in the English language from January 2000 through Oct 2019 that evaluated the method for identification of dental implants and its clinical importance for the dentist and the patient. The keywords included were: implant types, x-ray imaging, forensic, implant surface types, threaded, non-threaded, software identification, automated diagnosis. Some of the most relevant article’s full texts and reference lists were evaluated for eligibility.
Of the 387 articles found in initial search results, only 10 met the inclusion criteria set for this review. These 10 studies were directly related to the identification of different implant systems, which are presented in Table 1 . The relevant full-text articles and the reference lists of the related articles were evaluated to supplement the search as well. The assessment of the eligibility and finding related data were performed by two reviewers independently.
Upon analysis of the literature selected, different technologies for classifying and building a database for dental implants were found like the Implant Recognition Software (IRS), to identify implants in a person’s mouth, a method to identify threaded implants, non-threaded implants using radiographic images, a method to identify the design of selected dental implants, dental implant manufacturer database, a method to identify implants from Italy. The complications arising due to dealing with unidentified implants reiterating the need for a new system was found along with finding a potential solution in terms of a suggestion for companies to place individual serial numbers rather than batch numbers on the implants, and the filling out an implant record file by the patients to keep better tabs of the implants to build a database.
Identification technology for different implant systems
The articles and studies discussed in this scientific review are distributed as shown in Fig. 1 a. G. Michelinakis et al. ( 2006 ) created a webpage at Manchester University, United Kingdom, and collected all data available for root-formed implants obtained from the Google search engine ‘ www.google.co.uk ’ and the AltaVista search engine ‘ www.altavista.com ’, as shown in Table 2 . The webpage involved a detailed search of the World Wide Web (web) for implant manufacturing companies, with the initial search period being from November 2002 to June 2003 and updates commenced in February 2004 and ended in April 2004; a total of a 10-month search period. Implant types after April 2004 were not included. The data from this period was classified according to the implant type, body shape, implant design, abutment connection type, threaded or non-threaded, the surface type, polished collar, the diameter and length available for each system. The details of each implant system, according to each manufacturer, were then collected and stored in the IRS. This data, though collected from 21 different countries, produced a total of only 231 different implant designs. IRS ideally made it possible for the dentist and the lab workers to identify each dental implant system. However, because the IRS online tool was only updated during a limited time period, this system is no longer as beneficial as it was during that time.
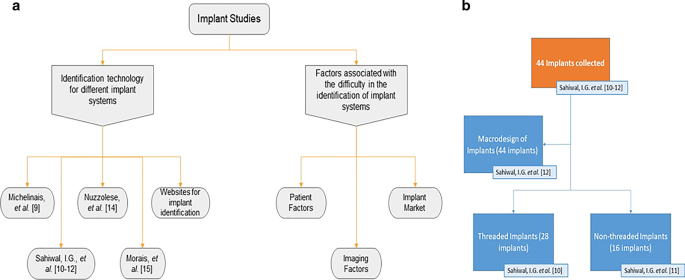
a Schematic of the studies involved in the review; b Three studies conducted by Sahiwal et al. (Sahiwal et al. 2002a ; Sahiwal et al. 2002b ; Sahiwal et al. 2002c ) describing different types of implants: Threaded and Non-threaded including the macro design of each
Another study by Sahiwal et al. ( 2002a ) documented various x-ray photos with different horizontal rotations and vertical angulations to the x-ray beam for each implant system, shown in Fig. 1 b. This study was for the threaded implants identification only. In this study, about forty-four implants “3.7 mm D*10 mm L” were collected, twenty-eight of which identified as threaded from more than fifty implant industry companies. Radiographic x-rays were taken in 0°, 30°, 60°, and 90° horizontal rotation with − 20°, − 10°, 0°, + 10°, and + 20° vertical inclination relative to the x-ray beam. This resulted in the production of twenty photos for each implant but at 20° vertical inclination. However, the x-ray photos were distorted and unrecognizable, so the observation was made only from − 10° and + 10° vertical inclination. They made tables describing each coronal part, middle part, apical part of each implant at vertical inclinations of − 20 to + 20, and − 10 to + 10 of the screw chambers. These tables were meant for the dental professional to match the description of their patient's implant x-ray to the tables provided in this study to identify what threaded implant they are working with. As for the non-threaded implants, Sahiwal et al. ( 2002b ) documented the features of different types of non-threaded dental implants in which they used the same protocol as described in the threaded study. More than fifty implant manufacturers were contacted, and out of forty-four implant that were donated, sixteen were non-threaded with "3.7 mm D*10 mm L" dimensions, as shown in Fig. 1 b.
Sahiwal et al. ( 2002c ) also studied the Macro design and the morphology of endosseous dental implants. In this study forty-four implants of size: "3.75mmD*10 mm" were donated and then classified into threaded and non-threaded, and tapered and non-tapered implants, as shown in Fig. 1 b. They examined each implant individually into 3 sections: coronal third, middle third, and apical third of the fixture. Then a table was formed describing each section of the fixture. This comparative method gives the dentist a database feature for each design and help in the radiographic identification for each system. However, as shown in Table 2 , the limitation of all three studies by Sahiwal et al. ( 2002a , b , c ) was that identifying the implants was cumbersome. These tables were limited to the forty-four implants that were donated and there is no software available making it prone to human error. This is not an up to date method and hence a more exact method is still required for more accurate identification of implants.
According to a study by Mansour et al . ( 2019 ), possibility of identifying the batch numbers, even if they were not engraved in dental implants, making antemortem dental records of dental implants more easily accessible to establish a comparative dental identification. In addition, the reported case presents the supplementary data yielded through estimating the epigenetic age using DNA (deoxyribonucleic acid) methylation as well as the biogeographical origin using Y-Haplotype and mitochondrial DNA analyses. Our results demonstrate that expanded oral implant investigations that also include implants extraction and comprehensive microscopic measurements can lead to identifying their batch numbers despite the numerous number of implants systems manufactured and distributed worldwide. Data saved by dental implant manufacturers can be very supportive and represent additional reference data for dental identification, when antemortem dental records are still missing.
Nuzzolese et al. ( 2008 ) studied the radiographic dental implants recognition for geographic evaluation within human identification. This study was carried out in Italy; the researchers created an archive of radiographic photos of Italian dental implants taken at horizontal rotations of 0º, 30º, and 60 º and combined with -20º, -10º, 0º, + 10º, and + 20º vertical inclination. They summarized the data into fifteen photos for each implant system. The observation was only in a -10º and + 10º vertical inclination; this study shows the survey of the distribution of the implant market over Italy thus give a clue of the geographic identification. However, the implant information was solely collected from Italy, geographically limiting this study.
According to Morais et al . ( 2015 ), a dental implant recognition novel computer-aided framework was suggested. They used this method for a segmentation strategy for semi-automatic implant delineation and a machine learning approach for the recognition of an implant model design. Although the segmentation technique was the focus of the recent study, preliminary details of the machine learning approach were also reported. Two different scenarios were used to validate the framework: (ACP 2020 ) comparison of the semi-automatic contours against implant’s manual contours of 125 x-ray images; and (NIH 2020 ) classification of 11 known implants using a large reference database of 601 implants. In experiment 2, 91% of the implants were successfully recognized while reducing the reference database to 5% of its original size. The segmentation technique achieved accurate implant contours. Although the results of the preliminary classification proved the concept of the current work it had limitations like the lack of detecting distinct features on implants; this software, though a step in the right direction, still needs an expansion of implants details in the database.
The current methods are limited to the dentists worldwide but not only does the dental field benefit from the identification of different implant system but also the forensic field gains significantly by classifying implant systems as it may provide the missing link to complete the picture (Bush and Miller 2011 ). For example, the identification of a disaster victims from their dental records is a well-established technique. In cases in which dangerous high temperatures from fires causes destruction of the structural integrity of the dentition, implants prove to be the only recognizable features. A study by Berketa et al. ( 2010a ) talks about how efficiency of implant recognition can be increased if the implant manufacturers were to place individual serial numbers rather than batch numbers on them. In another one of his studies (Berketa et al. 2010b ), he talks about how the Implant recognition software in its current form was of little benefit for radiographic assessment of dental implants for forensic odontologists. One way to improve this has been suggested by Daher et al. ( 2009 ) where they how an Implant Record Form filled by the patients would prove to be highly beneficial in keeping track of them.
Certain websites can be helpful in the task of identifying implants, as mentioned before. Sites such as whatimplantisthat (whatimplantisthat. 2020 ), Osseosource (OSSEOsource 2020 ), and Whichimplant (no longer available) are open source search engines that allow identification of implants through its radiographic photos. "Exotic encounters with dental implants: managing complications with unidentified systems" a case report by Mattheos et al. ( 2012 ), reported a 55-year-old male patient, with a dental implant from outside the country needed implant therapy. He identified his implant through these websites using his x-rays. A dental implant identification app was also launched three years after the conception of whatimplantisthat.com based on it (Kent Howell 2013 ). This app made its dental database easily accessible on the go to help better dental care provided by clinicians. In recent times, with the increasing utilization of artificial intelligence (AI) in various fields, a website which uses cloud-based AI to help dentist interpret x-ray images to find 30% more pathologies by specially developed machine learning algorithms (Tuzoff and Denti 2017 ). Though currently, the app and site whatimplantisthat.com is the most accurate and time-saving method, the AI website is a step in the right direction, and incorporating its featured to create an implant database will revolutionize this field.
Factors associated with the difficulty in the identification of implant systems
Implant market size and manufacture variety (fig. 2 a).
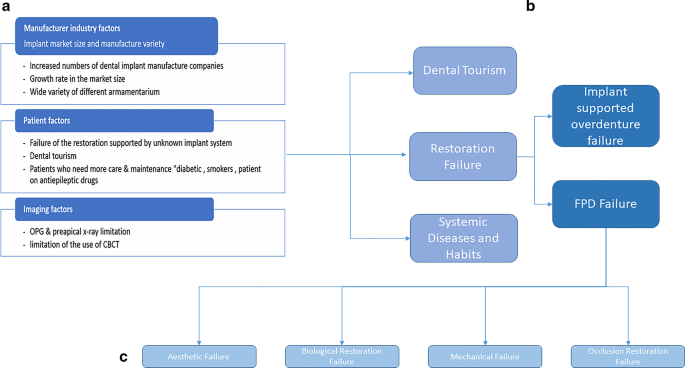
a Summarization of the factors associated with the complexity of implant identification. b Distribution of patient factors. c The failure types of implant-supported restorations
According to Dental Implants Statistics, the global dental implants market is likely to arrive at USD 13.01 billion by 2023 from USD 9.50 billion in 2018, at a CAGR of 6.5% (MarketsandMarkets™ 2023 ). The need for dental implants is growing; over 69% of Americans ages 34 to 44 years old have at least one tooth missing. More than 35 million people have an edentulous jaw or both (Gaille 2018 ). Additionally, according to the National Institute of Dental and Craniofacial Research, 24% of elderly people above 74 years old already have lost all their teeth (NIH 2020 ). During the period of 2014 to 2017, major players adopted product launches to strengthen their product portfolio and widen their customer base, followed by agreements, partnerships, and collaborations (Markets et al. 2023 ). Institute Straumann AG (Straumann) (Switzerland), DENTSPLY Sirona Inc. (DENTSPLY Sirona) (US), Zimmer Biomet Holdings, Inc. (Zimmer Biomet) (US), Danaher Corporation (US), AVINENT Implant System (AVINENT) (Spain), being the predominant players in the current dental implant market (Marketwatch 2024 ). The implants by various companies differ from each other subtly and accurate model is hard to detect even by an experienced eye. The difficulties with the identification of dental implants are increasing along with the rapid exponential growth of its market with the armamentarium for each system varying along with it.
Dental tourism: cost and age (Fig. 2 b)
Dental tourism is a major impacting factor that many clinicians are facing in identifying dental implant systems of each patient. There are many reasons why patients choose to receive implant restorations outside of the United States, first being the cost factor. 3–4 percent of the world’s population travels across borders receive healthcare; it is estimated that this industry is growing by 25 percent per year (Aircare Air Ambulance and Medical Escort Services 2020 ). It is estimated that 1.3 million Americans left the US for medical care in 2016; about 50 percent went to Mexico for dental procedures and another 15 percent traveled for cosmetic procedures (28). The ages of patients vary as well. Most statistics show that older patients are more likely to consider traveling for healthcare, but VISA surveyed over 30,000 people, 18 to 34 years of age, results pointed to about 88% of the total respondents made trips at least once per year, sometimes three times, for healthcare procedures (28). Statistics show that 400,000 Americans crossed international borders for dental care. According to a study, (Moeller et al. 2010 ), in 2016, uninsured low-income patients are less likely to receive dental services or may only have non-major dental care. The cost factor prevents most of uninsured patients to have dental treatment.
Restoration factors
Failure of the restoration supported by an unknown implant system (fig. 2 b).
Prosthetic failure may be due to an implant supported overdenture failure, FPD (fixed partial denture) failure, biological restoration failure, or an occlusion restoration failure. Failure of the restoration supported by an unknown implant system includes Peri-implantitis, restoration loosening, and fracture and implant structural damage. They may occur in implant-supported single or splinted crowns, bridges, overdentures, or fixed dentures (Gong 2018 ).
Implant supported overdenture failure (Fig. 2 b)
According to José Balaguer et al. ( 2015 ), overdentures were examined over 95 months (ranging from 36 to 159 months) with an overall success rate of 96.1%: 91.9% in the maxilla and 98.6% in the mandible, this being a significant difference (P < 0.05). Over the 13-year follow-up, 14 implants failed (3.9%), 12 due to peri-implantitis, and 2 due to implant fracture average duration of loading before failure was 52 months. The survival rate is not perfect, which implies that a dental professional may need to replace the overdenture leading to clinicians needing information pertaining to the implant system which is critical for accurately replacing the denture. According to Vahidi F et al. ( 2015 ), even with the success rate, implant-supported removable prostheses require episodic maintenance making it a critical factor and hence making the need for a systematic classification and database for implants indispensable irrespective of the need for replacement.
Fixed partial denture (FPD) failure
According to Bjarni E. Pjetursson et al. ( 2012 ), the meta-analysis of these studies indicated an estimated survival of implants supporting FPDs of 95.6% after 5 years and 93.1% after 10 years. The survival rate of implant supported FDPs was 95.4% after 5 years and 80.1% after 10 years of function. The success rate of metal-ceramic implant-supported FDPs was 96.4% after 5 years and 93.9% after 10 years (Sadid-Zadeh et al. 2015 ). Only 66.4% of the patients were free of any complications after 5 years. The most frequent complications in all implant supported restorations over the 5-year observation period is shown in Table 3 . These mechanical complications can be developed from even a single tooth restoration or fixed partial denture restoration supported by implants. Failure types of implant supported restoration are broadly shown in Fig. 2 c.
Aesthetic, biological restoration, mechanical and occlusion restoration failures (Fig. 2 c)
These two factors are correlated. Aesthetic failure due to gingival recession, which is a biological component, over an implant will affect the aesthetic aspect, especially in the front teeth. Aesthetic failures can be categorized as gingival failures or emergence profile failure and white-tissue failures (Fuentealba and Jofre 2015 ). According to Sailer et al. ( 2015 ), the feldspathic based porcelain should be limited to applications in the anterior region due to metal showing and zirconia ceramic crowns should not be considered as a primary option due to their high frequency of technical problems. Avoiding biological failures is critical in maintaining the health of the periodontium. The number of dental implants is increasing, a healthy peri-implant soft and hard tissues are required for the stability and survival of dental implants (Algraffee et al. 2012 ). Biological restoration failure includes the complication of peri-implantitis. The restoration itself can be lacking in the biological component (i.e. implant surface is rough). Daubert et al. ( 2015 ) found in his study that one in four patients and one in six implants have peri-implantitis after 11 years. According to Lee CT et al. ( 2017 ), peri-implant diseases were prevalent and the occurrence of peri-implantitis increased with time. According to Bergmann et al. ( 2014 ), dental implants are situated into an ever-changing environment in which teeth can continue to move around but the implants are ankylosed. Teeth may continue to erupt, leaving the implants in infraocclusion or move medially away from an implant which requires modification of the restoration. All these failures are components in which identifying the implant system is essential for everyone.
Systemic conditions and habits (Fig. 2 b)
The systemic conditions and the drugs used in the treatment of various conditions influence the implant restoration success rate; also, habits like smoking and bruxism have an impact on the success of the restoration. According to Manor et al. ( 2017 ), medically compromised people go ahead with surgery increasing failure rate. Certain medications involved in medical treatment can also be a factor. According to Chrcanovic et al. ( 2016 ), antidepressants are a statistically significant predictor for implant failure. Smoking is another factor as it is shown that implant restorations in smokers have a high failure rate, risk of postoperative infection, and marginal bone loss. With that said, smoking is not an absolute contraindication for implant treatment, but patients should be advised of the high risk of failure (Keenan and Veitz-Keenan 2016 ). Diabetes mellitus is also associated with a high risk of peri-implantitis, independently of smoking, but not with peri-implant mucositis, according to Monje et al. ( 2017 ). More research must be done because there is not yet a clear association between diabetes and implant failure (Naujokat et al. 2016 ). Bruxism may also significantly increase the implant failure rate and the complications of implant-supported restorations (Chatzopoulos and Wolff 2018 ). These conditions and habits hence affect the implant success rate and make their identification important.
Imaging factors (Fig. 2 a)
The problem in identifying an implant with a standard 2D (NIH 2020 ) x-ray is that 3D (Insights et al. 2027 ) spatial information is necessary for identification (Perlea et al. 2016 ). Additionally, the unknown implant insertion angle inside the jawbone, the horizontal rotation, the vertical inclination, and the direction of the x-ray beam were also contributing factors that need to be accounted for while photographing and documenting the data as it is important for the interpretation and identification of the implant x-ray photo as shown by Indira G. Sahiwal et al. ( 2002a , b ). Choi JW et al. ( 2011 ) confirmed that for a 3D x-ray, the use of CBCT (cone beam computed tomography—diagnostic aid used when the conventional x-rays fail as a diagnostic tool (Shah et al. 2014 )) should be preferred over a CT (computed tomography) image but it has a high radiation dose. According to Michele M Vidor et al . ( 2017 ), radiographic image of the bone-implant interface is influenced by two factors, the radiographic system, and the processing filter employed. The results from this study are that conventional radiographs or digital images with application of high-pass filters such as “Caries2” and “Endo” could help enhance diagnosis on the implant-bone interface on intraoral radiographs so it could help too in the identification of different implant systems and hence aid in the identification of different implant systems. Its flexibility with output formats, calibration of magnified images, and instantaneous results make it a highly efficient method (Gupta et al. 2015 ). According to Narra et al. ( 2015 ), Micro-CT was found to be a valuable tool for the morphologic evaluation of retrieved dental implants. Therefore, imaging factors influence the identification and indexing of current dental implants.
According to the growing rate of the implant market and the significant increase of the implant manufacturer's design, the identification of different implant systems has become a critical issue. Not only the growth of different implant designs has been deemed an issue but also the global increase in patients in need of treatment.
The maintenance factor needed in the post-implant treatment for every system is different within each armamentarium therefore; the clinicians and the lab technicians need to know exactly which system they are dealing with. Implants are a restoration that needs maintenance. Some patients who have medical conditions like diabetes and epilepsy are at greater risk for implant restoration failure. These patients need maintenance of periodontal health, which makes the identification for the implant restoration critical.
The development of a new and extensive database for implants is vital for successful implant therapies. The current technologies include limited databanks either due to lack of samples or geographical restrictions. Others are either with a limited period or only give 2D data where 3D information for implants are needed. Given the lacking current technology, keeping a tab on all the implants is very difficult and development needs to be made to design a more efficient, exhaustive, and accurate system.
The identification based on radiographic imaging needs more information about the horizontal rotation and vertical inclination of the fixture inside the jawbone. Digital radiography has shown to be highly effective in taking implant images due to its calibration of magnified images. Using digital imaging radiographic photos with high resolution and high pixelation is important to make the identification easier. Software programs or mobile applications based on documentation technology make it easier and more efficient for the dentist to use which saves significant chair side time and cost. More research is needed to cover the market variety and the update for the database is mandatory and essential for the awareness of dental implant global production.
Availability of data and material
The datasets used and/or analyzed during the current study are available from the corresponding author on reasonable request.
Abbreviations
American dental association
American college of prosthodontists
United States of America
European union
Implant recognition software
United Kingdom A
Deoxyribonucleic acid
Fixed partial denture
2 Dimensional
3 Dimensional
Cone beam computed tomography
Computed tomography
ACP. American collage of prosthodontics. Facts & Figures. https://www.gotoapro.org/facts-figures/ . Accessed 28 Aug 2020
Aircare Air Ambulance and Medical Escort Services . Top Dental Tourism Statistics for Dental Procedures Abroad 7/27/2017 [updated September 26, 2017. http://www.airambulanceone.com/medical-tourism-trends-facts-figures/ . Accessed 28 Aug 2020
Algraffee H, Borumandi F, Cascarini L (2012) Peri-implantitis. Br J Oral Maxillofac Surg. 50(8):689
Article PubMed Google Scholar
Balaguer J, Ata-Ali J, Penarrocha-Oltra D, Garcia B, Penarrocha-Diago M (2015) Long-term survival rates of implants supporting overdentures. J Oral Implantol 41(2):173–177
Bergmann RH (2014) Occlusal considerations for dental implant restorations. Compend Contin Educ Dent. 35(7):455–8
PubMed Google Scholar
Berketa JW, Hirsch RS, Higgins D, James H (2010b) Radiographic recognition of dental implants as an aid to identifying the deceased. J Forensic Sci 55(1):66–70
Berketa J, James H, Marino V (2010a) Survival of batch numbers within dental implants following incineration as an aid to identification. J Forensic Odontostomatol 28(1):1–4
CAS PubMed Google Scholar
Brandon Gaille. 17 Dental Implant Industry Statistics and Trends Aug 5, 2018. https://brandongaille.com/17-dental-implant-industry-statistics-and-trends/ . Accessed 28 Aug 2020
Bush M, Miller R (2011) The crash of colgan air flight 3407: advanced techniques in victim identification. J Am Dent Assoc 142(12):1352–1356
Cappare P, Sannino G, Minoli M, Montemezzi P, Ferrini F (2019) Conventional versus digital impressions for full arch screw-retained maxillary rehabilitations: a randomized clinical trial. Int J Environ Res Public Health 16(5):829
Article PubMed Central Google Scholar
Chatzopoulos GS, Wolff LF. Symptoms of temporomandibular disorder, self-reported bruxism, and the risk of implant failure: a retrospective analysis. Cranio. 2018:1–8.
Choi JW (2011) Assessment of panoramic radiography as a national oral examination tool: review of the literature. Imaging Sci Dent 41(1):1–6
Article PubMed PubMed Central Google Scholar
Chrcanovic BR, Kisch J, Albrektsson T, Wennerberg A (2016) Factors influencing early dental implant failures. J Dent Res 95(9):995–1002
Article CAS PubMed Google Scholar
Daher T, Goodacre CJ, Morgano SM (2009) Implant treatment record form. J Prosthodont Implant, Esthetic Reconst Dent 18(4):366–368
Google Scholar
Daubert DM, Weinstein BF, Bordin S, Leroux BG, Flemming TF (2015) Prevalence and predictive factors for peri-implant disease and implant failure: a cross-sectional analysis. J Periodontol 86(3):337–347
Dr. Kent Howell DMD MaDNFD, MS. Dental implant identification mobile app Nov 22nd, 2013. https://www.dentistryiq.com/dentistry/article/16353808/dental-implant-identification-mobile-app . Accessed 28 Aug 2020
Fox News. Dental tourism could save you big money 2013. https://www.foxnews.com/travel/dental-tourism-could-save-you-big-money Accessed 28 Aug 2020
Fuentealba R, Jofre J (2015) Esthetic failure in implant dentistry. Dent Clin North Am 59(1):227–234
Gong P (2018) Role of occlusion in complications of implant rehabilitation. Zhonghua Kou Qiang Yi Xue Za Zhi 53(12):800–804
Gupta S, Patil N, Solanki J, Singh R, Laller S (2015) Oral implant imaging: a review. Malay J Med Sci MJMS 22(3):7–17
Keenan JR, Veitz-Keenan A (2016) The impact of smoking on failure rates, postoperative infection and marginal bone loss of dental implants. Evid Based Dent 17(1):4–5
Lee CT, Huang YW, Zhu L, Weltman R (2017) Prevalences of peri-implantitis and peri-implant mucositis: systematic review and meta-analysis. J Dent 62:1–12
Manor Y, Simon R, Haim D, Garfunkel A, Moses O (2017) Dental implants in medically complex patients-a retrospective study. Clin Oral Investig 21(2):701–708
Mansour H, Sperhake JP, Bekaert B, Krebs O, Friedrich P, Fuhrmann A et al (2019) New aspects of dental implants and DNA technology in human identification. Forensic Sci Int 302:109926
MarketAnd Markets. Dental Implants and Prosthesis Market by Type (Dental Implants, Bridge, Crown, Abutment, Dentures, Veneers, Inlay & Onlays), Material (Titanium, Zirconium, Metal, Ceramic, Porcelain Fused To Metal), Type of Facility - Global Forecast to 2023 2019 https://www.marketsandmarkets.com/Market-Reports/dental-implants-prosthetics-market-695.html . Accessed 28 Aug 2020
MarketsandMarkets™. Dental Implants and Prosthesis Market by Type (Dental Implants, Bridge, Crown, Abutment, Dentures, Veneers, Inlay & Onlays), Material (Titanium, Zirconium, Metal, Ceramic, Porcelain Fused To Metal), Type of Facility - Global Forecast to 2023.
Marketwatch. Dental Implants Market : Global Trends, Share, Growth, Opportunity And 2024 2019. https://www.marketwatch.com/press-release/dental-implants-market-global-trends-share-growth-opportunity-and-2024-2019-11-15 . Accessed 28 Aug 2020
Mattheos N, Janda MS (2012) Exotic encounters with dental implants: managing complications with unidentified systems. Aust Dent J 57(2):236–242
Michelinakis G, Sharrock A, Barclay C (2006) Identification of dental implants through the use of implant recognition software (IRS). Int Dent J 56:203–208
Moeller JF, Chen H, Manski RJ (2010) Investing in preventive dental care for the medicare population: a preliminary analysis. Am J Public Health 100(11):2262–2269
Monje A, Catena A, Borgnakke WS (2017) Association between diabetes mellitus/hyperglycaemia and peri-implant diseases: Systematic review and meta-analysis. J Clin Periodontol 44(6):636–648
Morais P, Queirós S, Moreira AHJ, Ferreira A, Ferreira E, Duque D, et al. Computer-aided recognition of dental implants in X-ray images: SPIE; 2015.
NIH.National Institute of Craniofacial Research. https://www.nidcr.nih.gov/research/data-statistics/tooth-loss/seniors . Accessed 28 Aug 2020
Narra N, Antalainen AK, Zipprich H, Sandor GK, Wolff J (2015) Microcomputed tomography-based assessment of retrieved dental implants. Int J Oral Maxillofac Implants 30(2):308–314
Naujokat H, Kunzendorf B, Wiltfang J (2016) Dental implants and diabetes mellitus-a systematic review. Int J Implant Dent 2(1):5
Nuzzolese E, Lusito S, Solarino B, Di Vella G (2008) Radiographic dental implants recognition for geographic evaluation in human identification. J Forensic Odontostomatol 26(1):8–11
OSSEOsource. http://www.osseosource.com/dental-implants/ Accessed 28 Aug 2020
Perlea P, Nistor C, Suciu I (2016) Post-treatment periapical periodontitis X-ray versus CBCT - a case report. J Med Life 9(1):84–87
CAS PubMed PubMed Central Google Scholar
Pjetursson BE, Thoma D, Jung R, Zwahlen M, Zembic A (2012) A systematic review of the survival and complication rates of implant-supported fixed dental prostheses (FDPs) after a mean observation period of at least 5 years. Clin Oral Implants Res 23(Suppl 6):22–38
Premium Market Insights, Reports MDI. Dental Implants Market to 2027 - Global Analysis and Forecasts by Product (Dental Bridges, Dental Crowns, Dentures, Abutments, and Others); Material (Titanium Implants & Zirconium Implants); End User (Hospitals & Clinics, Dental Laboratories, and Others) and Geography Mar-2019. https://www.premiummarketinsights.com/reports-tip/dental-implants-market . Accessed 28 Aug 2020
Sadid-Zadeh R, Kutkut A, Kim H (2015) Prosthetic failure in implant dentistry. Dent Clin North Am 59(1):195–214
Saghiri MA, Saghiri AM (2017) In memoriam: Dr. Hajar Afsar Lajevardi MD, MSc, MS (1955–2015), Iran. J Pediatr 27:e8093. https://doi.org/10.5812/ijp.8093
Article Google Scholar
Sahiwal IG, Woody RD, Benson BW, Guillen GE (2002a) Radiographic identification of threaded endosseous dental implants. J Prosthet Dent 87(5):563–577
Sahiwal IG, Woody RD, Benson BW, Guillen GE (2002b) Radiographic identification of nonthreaded endosseous dental implants. J Prosthet Dent 87(5):552–562
Sahiwal IG, Woody RD, Benson BW, Guillen GE (2002c) Macro design morphology of endosseous dental implants. J Prosthet Dent 87(5):543–551
Sailer I, Makarov NA, Thoma DS, Zwahlen M, Pjetursson BE (2015) All-ceramic or metal-ceramic tooth-supported fixed dental prostheses (FDPs)? A systematic review of the survival and complication rates. Part I: Single crowns (SCs). Dent Mater. 31(6):603–23
Shah N, Bansal N, Logani A (2014) Recent advances in imaging technologies in dentistry. World J Radiol 6(10):794–807
site AAID. American Acadamy of Implant Industry. Available from: https://www.aaid-implant.org/faq/#267 Accessed 28 Aug 2020
Takeuchi Y, Koizumi H, Furuchi M, Sato Y, Ohkubo C, Matsumura H (2018) Use of digital impression systems with intraoral scanners for fabricating restorations and fixed dental prostheses. J Oral Sci 60:1–7
Article PubMed CAS Google Scholar
Tuzoff D. Denti.AI 2017. https://www.denti.ai/ . Accessed 28 Aug 2020
Vahidi F, Pinto-Sinai G (2015) Complications associated with implant-retained removable prostheses. Dent Clin North Am 59(1):215–226
Vidor MM, Liedke GS, Vizzotto MB, da Silveira HLD, da Silveira PF, Araujo CW et al (2017) Imaging evaluating of the implant/bone interface-an in vitro radiographic study. Dentomaxillofac Radiol 46(5):20160296
whatimplantisthat. https://www.whatimplantisthat.com/ #. Accessed 28 Aug 2020
Download references
Acknowledgements
This publication is dedicated to the memory of Dr. H. Afsar Lajevardi (Saghiri and Saghiri 2017 ), a legendary Pediatrician (1953–2015). We will never forget Dr. H Afsar Lajevardi’s kindness and support. The views expressed in this paper are those of the authors and do not necessarily reflect the views or policies of the affiliated organizations. The authors hereby announced that they have active cooperation in this scientific study and preparation of the present manuscript. The Authors confirm that they have no financial i nvolvement with any commercial company or organization with direct financial interest regarding the materials used in this study. The Authors confirm that they have nothing to disclose. MAS acknowledges being a recipient of the New jersey health foundation award.
Not applicable.
Author information
Authors and affiliations.
Department of Restorative Dentistry, Rutgers School of Dental Medicine, MSB C639A, 185 South Orange Avenue, Newark, NJ, 07103, USA
Mohammad Ali Saghiri
Department of Endodontics, University of the Pacific, Arthur A. Dugoni School of Dentistry, San Francisco, CA, USA
Newark, NJ, USA
Peter Freag & Jessica Eid
Department of Periodontics, Rutgers School of Dental Medicine, Newark, NJ, USA
Amir Fakhrzadeh
Sector of Angiogenesis Regenerative Medicine, Dr. Hajar Afsar Lajevardi Dental Material and Devices Group (ADMD), Hackensack, NJ, USA
Ali Mohammad Saghiri
You can also search for this author in PubMed Google Scholar
Contributions
Designed: MAS, PF. Conducted: MAS, PF, AF, Analyzed/interpreted data: MAS, PF, AF Wrote the article: MAS, JE. Proofed/revised article: MAS, PF, AF, JE. All authors have read and approved the manuscript.
Corresponding author
Correspondence to Mohammad Ali Saghiri .
Ethics declarations
Ethics approval and consent to participate, consent for publication, competing interests.
The authors declare that they have no competing interests.
Additional information
Publisher's note.
Springer Nature remains neutral with regard to jurisdictional claims in published maps and institutional affiliations.
Rights and permissions
Open Access This article is licensed under a Creative Commons Attribution 4.0 International License, which permits use, sharing, adaptation, distribution and reproduction in any medium or format, as long as you give appropriate credit to the original author(s) and the source, provide a link to the Creative Commons licence, and indicate if changes were made. The images or other third party material in this article are included in the article's Creative Commons licence, unless indicated otherwise in a credit line to the material. If material is not included in the article's Creative Commons licence and your intended use is not permitted by statutory regulation or exceeds the permitted use, you will need to obtain permission directly from the copyright holder. To view a copy of this licence, visit http://creativecommons.org/licenses/by/4.0/ .
Reprints and permissions
About this article
Cite this article.
Saghiri, M.A., Freag, P., Fakhrzadeh, A. et al. Current technology for identifying dental implants: a narrative review. Bull Natl Res Cent 45 , 7 (2021). https://doi.org/10.1186/s42269-020-00471-0
Download citation
Received : 21 October 2020
Accepted : 14 December 2020
Published : 06 January 2021
DOI : https://doi.org/10.1186/s42269-020-00471-0
Share this article
Anyone you share the following link with will be able to read this content:
Sorry, a shareable link is not currently available for this article.
Provided by the Springer Nature SharedIt content-sharing initiative
- Automated identification
- Dental implants
- Restorations

An official website of the United States government
The .gov means it’s official. Federal government websites often end in .gov or .mil. Before sharing sensitive information, make sure you’re on a federal government site.
The site is secure. The https:// ensures that you are connecting to the official website and that any information you provide is encrypted and transmitted securely.
- Publications
- Account settings
Preview improvements coming to the PMC website in October 2024. Learn More or Try it out now .
- Advanced Search
- Journal List
- Pharmaceutics

Latest Trends in Surface Modification for Dental Implantology: Innovative Developments and Analytical Applications
Francesca accioni.
1 Departamento de Química Orgánica y Farmacéutica, Universidad de Sevilla, 41012 Seville, Spain; [email protected] (F.A.); moc.liamg@599191olol (M.M.)
Juan Vázquez
2 Departamento de Química Orgánica, Universidad de Sevilla, 41012 Seville, Spain; se.su@ollebac
Manuel Merinero
3 Departamento de Citología e Histología Normal y Patológica, Universidad de Sevilla, 41012 Seville, Spain
Belén Begines
Ana alcudia, associated data.
The data presented in this study are available on request from the corresponding author.
An increase in the world population and its life expectancy, as well as the ongoing concern about our physical appearance, have elevated the relevance of dental implantology in recent decades. Engineering strategies to improve the survival rate of dental implants have been widely investigated, focusing on implant material composition, geometry (usually guided to reduce stiffness), and interface surrounding tissues. Although efforts to develop different implant surface modifications are being applied in commercial dental prostheses today, the inclusion of surface coatings has gained special interest, as they can be tailored to efficiently enhance osseointegration, as well as to reduce bacterial-related infection, minimizing peri-implantitis appearance and its associated risks. The use of biomaterials to replace teeth has highlighted the need for the development of reliable analytical methods to assess the therapeutic benefits of implants. This literature review considers the state-of-the-art strategies for surface modification or coating and analytical methodologies for increasing the survival rate for teeth restoration.
1. Introduction
Dental implants are most similar to natural teeth in their mastication and aesthetics; they are also biocompatible and require biocompatibility, masticatory feature, and aesthetic follow-up [ 1 , 2 , 3 ]. The American Association of Oral and Maxillofacial Surgeons estimated that two million implants are placed per year worldwide. The longevity of the population and the demand for cosmetic dentistry have led to their increasing use [ 2 , 4 ].
Implants are expected to have a 90% success rate after 10 to 15 years of implantation. However, between 5% and 11% of dental implants do not result in the required osseointegration in the maxillofacial bone. A startling phenomenon that has arisen from the widespread use of dental implants is the health issue related to peri-implant disease [ 5 ]. The failure of the long-term stability of the dental implant occurs because of biological (20% because of peri-implantitis, from microbial plaque or bacterial infections [ 6 , 7 ]), and mechanical causes, (stress shielding causing osteopenia and clenching–bruxism overloading interfacial bone [ 8 , 9 , 10 , 11 ]). Figure 1 shows a schematic representation of a modern implant.

Modern implant placed in oral cavity.
The goal of researchers has been to replace normal teeth function and prevent the peri-implantitis issues using dental implants made of novel materials that trigger the osseointegration processes. Figure 2 shows a clear increase implant research [ 2 ] from the Web of Science database. Data were obtained using filters with the keyword “peri-implantitis”, refined by review articles with the timespan 1990–2020.

Trend of dental implants research according to the Web of Science database.
Osteointegration is triggered by a cascade mechanism starting with interfacial reactions between the implant surface and the blood cells and connective tissue [ 12 , 13 ]. Bone trauma to place the implants creates a fibronectin rich blood clot pillar for the cells to form a new tissue [ 14 ]. Subsequently, osteogenic cells start to release mineralized collagenous substance between the implant and the host. Eventually, bone remodeling triggers new bone formation [ 15 ].
Peri-implantitis has been described in the World Workshop on the Classification of Periodontal and Peri-implant Diseases and Conditions as “a plaque-associated pathological condition occurring in tissues around dental implants, characterized by inflammation in the peri-implant mucosa and subsequent progressive loss of supporting bone” [ 16 , 17 ], which can results in treatment failure [ 18 ]. Peri-implantitis can lead to an irreversible, infectious illness generated by multi-factorial risks that are grouped into the following four categories: excessive mechanical solicitations, bruise of peri-implant tissue, corrosion and colonization of pathogenic microorganisms [ 19 ].
In this sense, five hundred bacteria live in symbiosis in the oral cavity and can colonize the implant when plankton bacteria adhere to the biomaterial by van der Waals or gravitational forces. Their flagella, pili and proteins form small aggregates of bacteria that secret polysaccharides and proteins for the formation of a biofilm. Biofilms plays a key role in the protection of bacteria colonization from immune system cells and antibiotics (e.g., antibiotics resistance) [ 20 , 21 , 22 , 23 , 24 , 25 ]. Moreover, bacteria can induce apoptosis, and/or activate a cascade of proinflammatory molecules, boosting the osteoclastogenesis process to bone resorption. Bacterial colonization and biofilm formation enhance the risk of implant loss via peri-implantitis [ 26 , 27 , 28 , 29 , 30 , 31 ].
This review includes a brief historical overview followed by 1-engineering strategies in dental implants; 2-coatings; 3-trends in analytical chemistry to cope with both osseointegration with the host bone tissue, and peri-implantitis issues. A graphical overview is presented in Figure 3 .

Graphical overview of the review.
The latest engineering performances regarding dental implants are discussed. The topographic and/or physicochemical modification of implant surfaces leads to the capacity for osseointegration. The second part on novel coatings is based on biomaterials with osteoconductive and/or antimicrobial activities such as killing organisms that adhere to the implant or reduce the microbes’ adherence because of its antifouling activity or release of drugs. The third part describes the latest trends in analytical chemistry that have supported dental implantology research to facilitate future methodological studies.
2. Historical Overview of Implantology
A historical overview starting in Egypt is presented in Scheme 1 . The need to replace natural teeth can be traced back to 2500 BC Egypt where seashells were anchored into human jawbone and stabilized with the use of gold wire. Famous archeological remains have shown that the civilizations in South and North America and regions of the Middle Asia and Mediterranean created artificial teeth using carved stone, shells, bones and gold more than 2000 years ago [ 4 ]. Moreover, ruins in Honduras had a fragment of a mandible with three shells carved into tooth shapes, confirming that the Mayan civilization had the earliest known examples of artificial substitutes, dating from about 600 AD [ 4 ]. Staple, subperiosteal, and blade vent implants represent the most successful designs of prosthodontic reconstruction that can be found in the early literature, made of noble or base metals. These were but affected by mechanical and biological failures [ 4 , 32 ].

Graphical historical overview.
In the 1700s, Dr. Hunter mentioned the possibility of relocating teeth from one human to another. During this time in Europe, teeth were extracted from the disadvantaged or from cadavers for allotransplantation [ 33 ]. In 1809, J. Maggiolo placed a gold implant into a tooth socket after extraction, but unfortunately an inflammation lead to failure [ 32 , 33 ]. Silver capsules, corrugated porcelain, and iridium tubes were some of the materials employed for dental implants [ 34 ].
In the 1930s, Dr. Alvin Strock became known for the successful treatment of shipboard periodontal issues with antibiotics, by provided anchorage and support for restoring teeth. He and his brother used Vitallium (a chromium-cobalt alloy) which at the time was considered a biocompatible material. Implant discovery continually increased during the 1900s and particularly during World War II [ 32 , 33 ]. However, the most important discovery occurred in 1952, when Dr. Per-Ingvar Brånemark, an orthopedic surgeon studying the bone-remodeling process in rabbit femurs, noticed that osseous matter could regenerate and attach to titanium [ 35 ]. He defined this phenomenon as osseointegration and used this concept for implant dentistry. In 1978, Dr. Brånemark presented a two-stage threaded titanium root-form implant that was fixed in his patients in 1965 and lasted for 40 years [ 36 , 37 ]. Brånemark Implants ® have had a great impact on today’s dentistry [ 35 , 38 , 39 ]. Many brand devices have been developed (e.g., TiOblast ® , Osseotite ® , Steri-Oss Etched ® , TiUnite ® , ITI-TPS ® , Laser-Lok ® , SLActive ® ) [ 2 ], in order to improve the effectiveness of the dental implants and their rate of success. Besides, recent developments including a variety of surface modifications for dental implantology have been made to cope with the fact of being biologically inert, such as acid etching/grit blasting, hydrogen peroxide or acidic treatment, alternative nitride, hydroxyapatite or metal-based coatings among others [ 40 , 41 , 42 , 43 , 44 , 45 ].
3. Engineering Strategies in Dental Implantology
The success of dental implants depends on factors such as mechanical overloading, implant-abutment connection design, implant geometry, implant position, bone density, surface finish material of the implant, and micro gap [ 46 ]. All of these factors have demonstrable effects on the implant’s integration to the bone tissue and the stress distribution at the bone-implant interface (stress-shielding phenomenon). Osseointegration between the bone and the implant is considered to be the critical factor that interferes in the implant survival rate. Low osseointegration or peri-implant bone loss may cause micro-mobility to the implant and lead to its consequent loss. A peri-implant bone loss of greater than 1 mm in the first year after implantation and greater than 0.2 mm in the following year is considered a failure of the dental implant [ 46 ].
Engineering techniques for manufacturing dental implants have played a key role in device design, surface topographies, uncomplicated insertion into the host osseous matter, biocompatibility and costs. Gaviria et al. considered that biocompatibility measures the degree of osseointegration, which correlates to the success rate over a period of time [ 4 ]. It is basically influenced by biomaterials composition, implant geometry and surface features [ 47 , 48 , 49 ].
3.1. Biomaterials Composition
The most important properties of an implant biomaterial are: the modulus of elasticity (e.g., 18 GPa for cortical bone); tensile, compressive and shear strength; yield and fatigue strength; ductility (e.g., 8% is needed for manufacturing requirements); hardness and toughness; surface tension and surface energy, and finally, surface roughness [ 50 ].
Metals, ceramics and polymers have represented the materials of choice for dental products. Polymeric materials have only been used for fabricating shock-absorbing components because of their low strength. Among ceramics, hydroxyapatite (HA) is the most used because of the great biocompatibility and capability to help the osteoblast activity due to its similar composition to the mineral structure of the bone. However, its low mechanical strength makes it only suitable as a coating material (i.e., plasma-sprayed coatings) [ 4 , 51 ], as discussed later. Something similar happens with bioactive glasses (BGs), a special type of glasses that induce the formation of HA when get in contact with body fluids [ 52 ].
Among metals, titanium and its alloys, Ti6Al4V and Ti-β, have been the most commonly used materials because they can osseointegrate. However, all these metals have and materials still have higher modulus compared to the cortical bone (20–25 GPa for cortical bone and 110 GPa for commercially pure titanium and Ti-β). Commercially pure (c.p.) Ti was used in the first Ti implant ever applied, the Brånemark Implants ® , but they often fail due to high stiffness. Current dental implants have been manufactured to reduce their Young’s modulus. Trueba et al. [ 53 ] evaluated the mechanical behavior of superficially modified porous c.p. titanium dental implants fabricated by conventional powder-metallurgy and space-holder techniques. A novel, feasible and repetitive protocol of micro-milling of the implant thread (before powder metallurgy sintering), as well as surface modification treatments (after sintering), have also been implemented. These techniques add porosity and surface roughness to the stiffness and yield strength of implants. Macro-pores concentrate stress locally, and may act as a barrier to the propagation of micro-cracks. Higher rugosity was observed for virgin implants obtained with spacer particles. Concerning the superficial modification of implants, while BG 1393 was the most effective coating due to its greater infiltration and adhesion capacity, chemical etching could improve osteoblast adhesion because it modifies the roughness of the implant surface. Therefore, a new reliable protocol was developed and evaluated to fabricate titanium implants with improved biomechanical and biofunctional response of the interface with the host.
Lascano et al. [ 54 ] investigated the use of a Ti-β alloys, composed of Ti-Nb-Ta-xMn (x: 2, 4, and 6 wt%) and with a lower elastic modulus compared to the c.p. titanium. They included controlled porosity in the implants to reduce more their Young’s modulus, since it was still high (ranging from 50 to 60 GPa). In addition, they include a graphene layer onto the substrates’ surface to enhance their biocompatibility and cell adhesion. The alloy containing 4 wt% Mn favors the presence of the Ti-β phase, and Young’s modulus (8–9.3 MPa) closer to the trabecular bone. Furthermore, Ti6Al4V was developed by the aerospace industry but was applied to biomedical systems for its strength, corrosion resistance, and biocompatibility [ 55 ]. These implants have been fabricated in a variety of shapes, such as cylindrical, cone, hollow and screw shapes, and various diameters. Additionally, Ti6Al4V has also been used in orthodontic implants [ 56 ], which are temporary anchors. However, some authors reported its long-term toxicity with human osteoblastic and fibroblastic cells [ 57 ]. New research approaches tend to avoid the use of elements in the titanium alloys with the potential to cause tissue damage, such as vanadium [ 58 ].
Alternatively, zirconium and gold have also been used for the same purpose but, unfortunately, they have demonstrated poor bone-to-implant adhesion [ 4 ]. Other materials that have been used for the applications of titanium-based materials in dental implants are based on stainless steel, Co-Cr, Co-Cr-Mo-Ni-Fe or other metal combinations alloys where issues such as cytotoxicity, mechanical, chemical, electrochemical, or biological properties were addressed [ 59 , 60 , 61 , 62 ].
3.2. Implant Geometry and Surface Features
Implant geometry modulates the bone–prosthesis interaction and distribution forces, changing the surface area and the long-term stability. Thus, cylindrical screw-threaded implants are the most commonly placed [ 63 ].
Surface topography is a very important aspect to consider when designing and manufacturing a dental implant since it directly influences its bioactivity, that is, some of the parameters related to the implant’s survival rate such as the osseointegration or the appearance of bacteria-related infections. The mainstream engineering strategies used to obtain bioactive topographies with surface modifications of the implants are sandblasting, acid-etching, anodization, plasma spraying, and laser radiation ( Figure 4 ).

Mainstream engineering strategies in dental implantology.
These techniques have changed the free surface energy, chemical composition, and roughness, which may enhance osseointegration. Rough surfaces (e.g., S a value around 1-2 µm) within hydrophilic composition act as guide locomotion and cellular basement for the adhesion and trigger proliferation of osteoblasts, but high surface roughness can promote the development of peri-implantitis [ 46 , 64 , 65 , 66 ] due to bacterial proliferation.
A special mention is made to nanoengineering. The development of nanotechnology, nanoscale modifications and the application of nanomaterials have notably reduced the well-known key issues related to dental implants, such as low osseointegration, the stress-shielding phenomenon and implant-related infections. Nanotechnology has facilitated the implementation of surface modifications, the use of coatings and even the controlled release of antibiotics or proteins. The combination of these approaches has entailed the enhancement of the osseointegration and soft tissue integration of dental implants, as well as their antibacterial and immunomodulatory functions [ 64 ].
3.2.1. Sandblasting
Sandblasting implant surface requires particles of various diameters and are one of the most currently commercialized methods for surface modification with the advantages of both topography and wettability [ 66 , 67 ]. The technique of pressured air steam applied to the titanium implant surface generates a macro-roughness due to the projection of accelerated microspheres of TiO 2 , Al 2 O 3 , SiO 2 , or HA. Besides, these four chemical groups act with the same strength as boosting agents for osseointegration [ 68 ]. An interesting 4-year clinical observation study showed that titanium implants modified by the sandblasting approach had a higher overall clinical outcome [ 69 ]. However, a 20-year follow-up compared a non-modified turned surface device and TiOblast ® and suggested these topographies did not improve the bone healing but increased peri-implantitis and implant failure [ 39 ].
During the sandblasting process, when abrasives are projected to the surface, in order to obtain the optimum macro-roughness, some factors must be monitored [ 70 ]. In particular, the choice of the employed particles (i.e., type, size and shape) is a key point, and abrasives must be harder than implant materials to produce roughness [ 70 , 71 ]. Moreover, the distance from the projection gun to the surface, the projection pressure, the saturation time and projection diameter represent important parameters that influence the roughness [ 70 ]. Sandblasting has been considered a key treatment to modify implant surfaces with osseo-inductive activity, and to increase the contact angles for the improvement of hydrophilic behavior for osteoblasts adsorption [ 70 , 72 ]. On the other hand, sandblasting induced by abrasives can lead to microbial contamination with the consequence of implant failure [ 72 ].
3.2.2. Acid-Etching
Osseotite ® (Zimmer Biomet, Warsaw, IN, USA) and Steri-Oss Etched ® (Nobel Biocare, Zürich-Flughafen, Switzerland) are commercial implants manufactured using the acid-etching strategy [ 73 , 74 , 75 ]. This technique produces micro-pits surfaces via the immersion of the metallic core in corrosive acids such as HCl, H 2 SO 4 , HNO 3 , and HF [ 76 ]. Commonly, the acid-etching erosion takes place after sandblasting and the whole process is considered as the reference surface treatment, referred to as sandblasting and large grit acid etching (SLA) [ 77 ]. The Osseotite ® demonstrated a success rates of >96% in long-term studies [ 73 , 74 ].
Other authors attempted an acid treatment with a piranha solution—a mixture 3:7 of H 2 O 2 (30 vol. %) and H 2 SO 4 (70 vol. %)—not only to confer roughness and therefore, the enhancement of osseointegration to the implant surface, but also to improve its antimicrobial capacity by the chemical linking of silver nanoparticles [ 78 ].
Surface features can be tailored by modifying the type and the concentration of the acids used for the acid-etching procedure. Besides, the time of exposition and the working temperature can affect the erosion with the formation of cavities of different dimensions [ 79 ]. Titanium surfaces exposed to acid-etching process have demonstrated positive results related to an increase in the roughness and osteogenic response due to the proliferation, adherence, and differentiation of osteogenic cells [ 80 , 81 , 82 ]. However, in cases of an uncontrolled process, with over-etching or under-etching of the surface, an important variation in mechanical properties, corrosion resistance, or biocompatibility have been observed [ 83 ].
3.2.3. Anodization
Anodization is an electrochemical technique used for the oxidation of titanium surface that improves biocompatibility, blood-clot formation, cell adhesion and osteoblast proliferation. During the process, the implant is immersed in acids such as H 2 SO 4 , H 3 PO 4 , HNO 3 while a current is applied, creating micropores that increase the oxide layer of TiO 2 in form of anatase. Anatase and rutile have been considered the most important TiO 2 phases. In particular, in the anatase phase, the unit cell is formed by four TiO 2 units, where O atoms are coordinated to three titanium atoms and positioned in the same plane in order to form unrelaxed structure [ 84 ]. TiUnite ® (Nobel Biocare, Gothenburg, Sweden) have been reported to present optimum results, and only 8.2% of the implants in the study were affected by peri-implantitis [ 85 ], due to the improvement of the osseointegration.
Anodic electrochemical oxidation is a technique capable of modifying oxide properties, depending on the working electrochemical parameters (e.g., applied voltage, electrolyte composition and concentration, anodization time, bath temperature, stirring) [ 86 ]. In fact, the ultrastructural level topographies are a consequence of the migration of the ions throughout the oxide layer, and the thickness of the anodic oxide is determined by Faraday’s Law. The anodization of dental implants was demonstrated to favor blood-clot retention [ 87 ], nano-roughness, and osseointegration [ 88 , 89 ]. However, despite the advantageous biological outcomes, the mechanical stability of implants exposed to anodization has represented a challenge to be addressed by many researchers [ 90 ], even if good clinical outcomes have been reported [ 87 ].
3.2.4. Plasma-Spraying
Plasma-spraying treatment can be associated with SLA to improve biocompatibility and protein absorption because of the additional layer of OH groups. In fact, the implant surface can be modified by the projection of titanium particles injected into a plasma torch at high temperatures, and a film about 30 µm thick is formed by Ti-OH residues [ 76 , 91 ]. ITI-TPS ® (Straumann Institute, Waldenburg, Germany) belong to this type of manufacturing in the market [ 92 ].
A plasma-spraying process embraces a thick layer of deposition. Hydroxyapatite and titanium are the most commonly used; they are thermally melted and afterwards sprayed on the implant surf area [ 93 ]. The combination of hydroxyapatite coating on titanium alloys has been considered very interesting because it demonstrated great biocompatibility and good mechanical properties [ 94 ]. Moreover, various strategies have been evaluated in order to obtain the adhesion of hydroxyapatite to titanium implants [ 95 ], but only plasma spraying has been actually used in the market [ 91 ]. However, this type of coating has demonstrated some drawbacks related to a reverse effect [ 93 ], since the bonding strength of hydroxyapatite on titanium alloys decreased by passing the time when the implant is immersed in the simulated body fluids [ 96 ]. Recently [ 97 ], a suspension plasma spray (SPS) was successful applied for the deposition of hydroxyapatite /gray titania coatings on a titanium surface. Authors reported an improvement in antibacterial properties due to an increase in the number of Magneli phases. Besides, an improvement in the bacterial adhesion was demonstrated because of the hydrophilic properties which correlate to the obtained submicron-sized particles.
3.2.5. Laser Ablation
The above-listed methods can facilitate the formation of macro and microroughness surfaces, while laser radiation creates a nano-topography modification. Laser ablation utilizes the laser sources to produce on-site melting of the metal, as a result of the heating induced by the absorption of the radiation [ 98 ]. Thus, a micromatching is formed by the presence of microchannels, prompting a faster healing of the bone [ 99 , 100 ]. This technique can adapt the light frequency, and take advantage of the high energy density and of the all frequencies available. Besides, it is possible to pulse the source and control the reaction time for obtaining the microstructures with increased hardness and corrosion resistance [ 48 , 101 ].
An example of an implant based on this treatment is Laser-Lok ® (BioHorizons, Birmingham, AL, USA) [ 102 ]. SLActive ® (Straumann Institute, Basel, Switzerland) also presents a nanorough surface carried over by blasting and acid etching with a final manufacturing step in which it is flushed with a stream of nitrogen, safeguarding the implant from air and then immersed into a NaCl solution for storage. The benefits of this type of technology include an increase in hydrophilicity in the performance of the biological response and cell recruiting, and faster healing of the bone, with a long-term success rate of around 91.7% [ 100 , 102 ].
Other authors have used femtosecond laser [ 103 , 104 ] or directed irradiation synthesis (DIS) [ 105 , 106 ] techniques to obtain micro- and nano-textured surfaces of titanium implants to improve osseointegration by the replication of bone structures, increasing protein attachment and therefore cell adhesion.
Laser ablation has also been used to generate antimicrobial surfaces. Thus, for example, Boutinguiza et al. [ 107 ] used this technique to deposit silver nanoparticles on top of c.p. titanium implants.
4. Coatings
The biocompatibility, osseointegration and therapeutic/antibacterial effect of the implant coating have been considered to be the most important factors for the long-term durability of dental implants. Various bioactive materials and local drug delivery techniques have been explored. These novel coatings have been divided into two groups, depending on the nature of the material applied ( Figure 5 ), i.e., organic and inorganic coatings.

Novel coatings in dental implantology.
Bioactive coatings onto core biometals synergize the bone-healing ability of a bioactive material and the leads to biomechanical advantages of a matrix such as porous titanium. The porous structure focuses stress restrictedly, and it circumscribes small-cracks. HA, magnesium, graphene, grow-factors/specific bone morphogenetic proteins (BMP), and BGs are materials used in functional-coatings technology. The aim in this case is to improve cell–proteins adhesion, mineralization of the implant–host interface, and antimicrobial behavior of the surface layer [ 53 , 108 ].
4.1. Inorganic Coatings
Nanostructured calcium, calcium phosphate, and HA) have been the most used implant coatings. They can be applied to a metal implant by hydrothermal deposition or the plasma spraying. These materials release calcium and phosphate ions to encourage the mineralization of the interface tissues and bone-healing [ 2 ]. Inorganic coatings also affect how stress is transmitted to the bone during masticatory function and the correct distributions of the forces during the repeated cycles [ 2 ].
4.1.1. Hydroxyapatite-Based Biomimetic Coatings
Biomimetic surfaces promote osseointegration in the healing process since they possess a composition similar to the host. HA is the stable biological mineral form of calcium apatite. It camouflages the mineral bone phase and triggers osteoblasts activity for bone formation. HA presents some unique properties because of both its non-inflammatory and non-immunogenic nature. The bioactivity can be boosted by using a micro-arc oxidation technique that allows the formation of a porous HA-coated surface of titanium alloy with positive consequences on biomechanical properties and bone formation [ 108 , 109 ].
Osstem GS-HA III ® and Osstem TS III-HA ® (Osstem Implant Co., Busan, Korea), which are HA-based devices, have been analyzed and they demonstrated a good balance in the transmission of these forces, but a second work, carried out by the finite element method (FEM), found them to have a weak performance over a long-term period. In spite of that, HA-based coatings showed an optimum rate of success (100%) with the presence of peri-implantitis in 2.2% of Osstem and TS III-HA ® implants and, in 1.4% of TSV-HA ® [ 2 ]. However, it was reported that the plasma-spraying strategy employed for obtaining some HA coatings on metallic implants have presented poor long-term adherence to the core, difficult reproducibility and a variability of the layer thickness and composition and, in addition, can lead to bacterial infections [ 32 ]. Other studies highlighted the bioactivity of biomimetic coatings for the reduction of the time required for osseointegration because of both the high wettability of the coated surface and the improved protein adsorption property [ 109 ].
Nanotechnology has been applied to HA particles in order to obtain a single coating and combine its bioproperties to collagen, BGs and titanium dioxide for making a biomimetic osteo-matrix. The nanoscale promotes both the specific surface area and adsorption feature, resulting in a better interaction with the host bone [ 110 ].
A HA coating deposited on metallic pins, which are metal devices used as support of dental amalgam for restoring the tooth [ 111 ], has also been studied as bioactive carrier surfaces for the release of an aminoglycoside antibiotic for antibacterial purposes. The tobramycin loaded on the biomimetic coating showed bactericidal activity against Staphylococcus aureus in an agar medium that longed 6 days [ 112 ]. Other authors incorporated the HA in a polymeric blend to obtain a composite coating with this antibacterial effect. Thus, García-Cabezón et al. [ 113 ] deposited HA-containing chitosan nanofibers onto porous titanium implants. The use of this composite to cover the implant’s surface would not only increase the osseointegration but would also prevent the corrosion of the implant. In addition, the demonstrated antibacterial behavior of chitosan and the inclusion of silver nanoparticles would avoid bacteria-related infections.
4.1.2. Calcium Phosphate-Based Biomimetic Coatings
After the implantation of calcium phosphate-based implants, Ca and P are released into the host tissues promoting the in locus formation of the apatite layer [ 114 ]. Enhanced clinical outcomes of implants based on calcium phosphate coatings, with a long-term success rate due to both the promotion of new tissue formation and a wider bone-to-implant contact surface, have been reported over the last years [ 115 , 116 ].
The presence of an inorganic nanotopography in the implant surface affects the cell behavior during the healing process by increasing the gene expression of osteogenic factors, mineralization, adhesion and proliferation. In fact, the coatings of Ossean ® (Intra-Lock, Boca Ratón, FL, USA) implants, fabricated by the impregnation of the calcium phosphate nanoparticles within surface microroughness due to a grit-blasting/acid-etching strategy, demonstrated an up-regulation of osteogenesis-related genes since the first week after the implantation and of alkaline phosphatase (ALP) kinase expression (a adhesion/proliferation-related gene) and higher minerals deposition than the dual acid-etched implants [ 117 , 118 ].
Another way to employ calcium phosphate nanoparticles in implant coatings has been represented by combining the acid-etching technique with discrete crystalline deposition (DCD) that allows for the impregnation of the inorganic part during the sol-gel process [ 119 ]. Clinical studies have demonstrated that these types of coatings promote osteoconduction with the implant and quite acceptable long-term durability with low bone resorption [ 120 , 121 , 122 ]. The bone-to-implant contact value demonstrated by Nanotite ® (Zimmer Biomet, Palm Beach Gardens, FL, USA), a calcium phosphate nanoparticle-based implant produced using the DCD method, demonstrated important differences in mechanical properties with micro-topographies systems. Micro-roughness implants presented a significantly higher removal torque value than DCD devices but better anchorage to the host bone [ 122 , 123 ].
4.1.3. Magnesium-Based Biomimetic Coatings
The biomimetic property and biodegradable nature of Mg alloys have been explored by researchers over the last few years for the fabrication of new biomaterials that resemble the human bone. Actually, phosphate salts of Mg have demonstrated better resorption kinetics and dissolution rates than calcium salts in clinical trials [ 124 ]. In vitro and in vivo studies evaluated this bioactivity which resulted in a better promotion of osteoblasts function and faster bone formation, in comparison to calcium phosphate surfaces, respectively [ 125 , 126 ].
Nevertheless, its alloys have shown some drawbacks with regard their short-term corrosion on the implant interface. Microwave-assisted preparation of magnesium phosphate crystals for obtaining coatings had good outcomes even if the mechanism behind this interaction technique is still under evaluation [ 127 ]. Besides, MgCl 2 and epigallocatechin gallate were employed as a metal–polyphenol network and for osteoinductive coating onto a titanium implant [ 128 ]. Moreover, a SLM (selective laser melting) process has been applied to produce porous titanium implant 3D surface with calcium phosphate and doped with magnesium to encourage bone formation. Results demonstrated a higher bone volume increase with magnesium-doped HA coatings than for the control group [ 129 ].
In addition, other researchers reported that nanoparticles of magnesium have been studied in dental implantology due to the high level of antibacterial activity against the biofilm formation in Staphylococcus implant infections [ 130 ].
4.1.4. Graphene-Based Biomimetic Coatings
Graphene application to coatings has been introduced as a new player in dental implants, also because it was isolated in 2004 from the exfoliation of graphite [ 131 ]. Graphene is a two-dimensional, one-atom-thick sheets of sp2-bonded carbon atoms, shaped in a hexagonal network. This unique make-up results in some important features such as great electronic, optical and mechanical properties. In fact, the graphene structure presents stability and resistance to degradation caused by mechanical or chemical stresses, differing from Ti6Al4V implants [ 132 ].
Lately, implant coatings have been developed based on graphene oxide (GO), or reduced graphene oxide, the two-dimensional nature of GO nanomaterial makes it possible to functionalize the large number of oxygen-containing groups on the active surface, with carboxyl and hydroxyl residues [ 133 ]. The ultrasonic atomization spraying process has been applied to coat an SLA titanium surface with GO, which showed a good stimulation of cell proliferation and adhesion and effect of the vascular endothelial growth factor on osteoblasts [ 134 ]. The coating formed by graphene/HA and deposited on the titanium implant presented a biomimetic behavior due to a better biocompatibility/activity and higher adhesion in comparison to coatings made only by calcium phosphate layer or titanium [ 135 ].
Moreover, the broad-spectrum antibacterial activity of graphene has been demonstrated by different studies on multi-resistant bacteria and fungi species, and the mechanism behind explored. The effect was due to the damaging of cell membranes through oxidative stress against the microorganisms [ 136 ]. In another study, thin layers of GO, incorporated with silver nanoparticles and coated onto the metallic core, showed activity against Streptococcus mutans and Porphyromonas gingivalis [ 137 ]. In addition, it has been demonstrated that the number of GO layers increases antibacterial and osteogenic activities, as shown by the minocycline-loaded GO implant that killed Staphylococcus aureus , Streptococcus mutans and Escherichia coli with no side effects on the human gingival fibroblasts [ 138 , 139 ]. In dental implants, GO hybrid materials have been tested using different concentrations of GO soaked in chitosan. These hybrid materials were deposited onto Ti substrates, demonstrating osteoblast proliferation and biofilm inhibition [ 140 ]. In Y-ZrO 2 implants, GO coatings improved the mechanical properties of samples, such as the bending strength and the fracture toughness, while increasing cell adhesion, proliferation and growth [ 141 ].
4.1.5. Carbon Nanotube-Based Coatings
Carbon nanotubes have gained momentum as biomaterial for medical applications since they present both chemical durability and biomechanical and electrical properties. They can be used as coatings for titanium cores covered with collagen, promoting surface roughness, cell proliferation and adhesion [ 142 ]. Moreover, the bone healing activity of these coatings has been well demonstrated by culturing multiwalled carbon nanotubes on rat primary osteoblasts. Alkaline phosphatase activity and calcium and osteopontin contents were higher than the non-coated group of the control. Eventually, after 28 days of the implantation of multiwalled carbon nanotubes with osteoblasts, the presence of the bone matrix was shown in the pores of the honeycomb structure [ 143 ]. Recently, an in vitro evaluation of multiwalled carbon nanotube reinforced nanofibers for dental application was performed. Multiwalled carbon nanotubes were incorporated into electrospun nanofibers of nylon-6 that were later added to a polymeric resin in different concentrations. The most promising composite was the one containing 2.5 or 5% of nanofibers due to their adequate flexural strength associated with reduced film-thickness [ 144 ]. In order to solve some drawbacks related to HA coatings, researchers have proposed HA-carbon nanotube composite coatings on a titanium core, obtained by aerosol deposition. These systems showed no microcracks, and led to an improvement in mechanical properties such as hardness and the elastic modulus. Besides, they presented a better clinical outcome by promoting cell proliferation and the alkaline phosphatase activity than coatings made only of HA and titanium [ 145 ]. Kim et al. demonstrated that the addition of carbon nanotubes to polymethyl methacrylate resins prevents bacterial adhesion without cytotoxicity to oral keratinocytes [ 146 ].
The nanoscale size and the large surface areas of carbon nanotubes and graphene have favored their use for making biomaterials for clinical purposes and regenerative medicine. However, since they interact with proteins, nucleic acids and host cells, their cyto-compatibility has been explored during the last few years and it is still controversial [ 147 ].
4.1.6. Nanodiamond Coatings
Nanodiamonds are formed by a unique structure and surface, containing oxygen-carrying groups and charges that have been considered responsible for the antibacterial activity of the coatings (e.g., Escherichia coli ). The biocompatibility of nanodiamonds-based coatings has been evaluated since their interaction with biological living organisms. A study of this type was presented by Vaitkuviene et al. [ 148 ] who observed the effects on neural cells, concluding that the thin layer of oxygenated and boron-doped nanocrystalline diamond increased cellular proliferation and adhesion. In general, particles a size of 100 nm or more presented better results than smaller ones. In addition, the current literature determining the mechanical properties and bond strength of nano-diamond coatings on dental implants is scarce and requires further research. In addition, the biocompatibility of nanodiamonds has been explored by in vivo studies, but controversial results have been obtained, and further investigations are required. In dental implantology, the literature is still incomplete concerning mechanical properties or the bond strength of the coating to the implant [ 109 ].
4.1.7. Silver-Based Coatings
Since the inorganic nature of silver as element, its activity as antibacterial agent was introduced in this section. Inorganic-based coatings with an effect against microorganisms have demonstrated better properties such as chemical stability, thermal resistance and long-lasting activity, than common organic agents. Besides, silver has exhibited oligodynamic antimicrobial activity that consists of bactericidal-bacteriostatic effect at very low concentrations. This agent has proved a broad spectrum of action against Gram-positive and Gram-negative bacteria within a low propensity to develop bacterial resistance, and a successful inhibition against the polymicrobial colonization [ 149 ]. The biocidal effect of silver is mainly due to Ag + ions which alter the permeability of cell walls, interfering with vital proteins and triggering DNA condensation ( Figure 6 ). This behavior has allowed silver-based coatings to be effective against the typical dental implants-associated pathogens (e.g., Streptococcus mutans , Staphilococcus aureus , Streptococcus Oralis , and Actinobacillus actinomycetemcomitans ).

Synthesis and proposed mechanisms of action of Ag + and silver nanoparticles in bacteria.
Antimicrobial and osteogenic properties of Ag + -implanted stainless steel have been explored with in vitro and in vivo tests. The silver-sourced plasma immersion ion implantation (Ag-PIII) was used for modifying the surface of stainless steel, which was doped with silver nanoparticles. The Ag-PIII treatment promoted anti-infective activity and demonstrated the osteogenic differentiation of bone marrow stromal cells [ 150 ]. The antimicrobial effect has also been explored for coatings made of HA–silver, plasma sprayed silver-implanted HA and silver loaded gelatin microspheres, incorporated into porous titanium that controls the metal release in situ [ 149 ].
The need for performing silver coatings by functionalizing porous titanium surfaces is based on their high rate of fail after implantation, mainly due to the presence of infections. Silver nanoparticles have been proposed as an important alternative to the common silver coatings since they induce a convenient prolonged release of Ag + ions. Moreover, silver nanoparticles are adsorbed onto the bacteria membrane, which is dependent on Coulomb gravity, triggering protein coagulation [ 151 , 152 ] ( Figure 6 ). A combination of a porous titanium implant, for the reduction of the stress-shielding phenomenon and enhancement of osseointegration, and a silver nanoparticle-coated substrate, for bacterial inhibition, have been tested. The deposition of nanoparticles was achieved by chemical bounding after a silanization reaction to the hydroxylated surface. In order to evaluate the outstanding properties of the implant, microstructural features and anti-infective effects against Staphylococcus aureus were considered [ 78 , 153 ]. Other authors highlighted the importance of a relevant nanocomposite coating for the antimicrobial properties by exploring the activity of surfaces formed of polysaccharide 1-deoxylactit-1-yl chitosan and silver nanoparticles on methacrylate thermosets. Moreover, the adequate level of ions content, delivered at first, was able to kill pathogens quickly and prevent the development of drug resistance.
However, over the topic remains controversial. On the one hand, the inactivation of the silver antimicrobial effect in human fluids and, on the other hand, the low cut-off level of Ag + ions required for cytotoxic activity, are very important aspects in the case of nanoparticles since the release is prolonged. Even so, the capability of silver as a biocide in absence of cytotoxic effect on osteoblasts and epithelial cells has also been demonstrated [ 154 ]. Silver electrodeposited on tricalcium phosphate (TCP)-coated titanium implants have been reported for the expected bactericidal effect without cytotoxicity since the optimal silver release. In the same way, the cold-spray coating technique utilizing polyether ether ketone (PEEK) and the chitosan–copper composite for creating antimicrobial implant coatings have presented similar results [ 109 ].
4.1.8. Bioactive Glasses
BG for metallic substrates coating has been considered, in comparison to other surface modifications, more effective and efficient in terms of a high Young’s modulus (preventing the stress-shielding phenomenon) and osseointegration (stimulating bone regeneration). In fact, BGs have indicated unique properties that provide a versatile tool, based on the possibility to change their composition, for clinical application, such as bone regeneration and drug delivery. Professor L. L. Hench gave the definition for BGs as “special glasses able to bond to bone or even to soft tissues, without any rejection” [ 52 , 155 ]. In general, they have demonstrated the capability to form precipitates of HA on the glass substrate ( Figure 7 ), when exposed to contact with human fluids, resulting in bonding to the host bone. The biomimetic ability to produce HA resembling the natural one has been demonstrated to promote the osseointegration of the implant. Besides, they also showed osteoinduction properties within the bone formation at the implant–device interface [ 156 ]. BG porosity and roughness, modulated by deposition techniques, are important features since they affect bioactivity. Moreover, the chemical composition, which is highly variable, is a key factor determining their final properties. According to it, they can be classified into different types, such as silicate-, borated- and phosphate-based BG coatings [ 157 ]. Silicate-based BGs (e.g., BG 45S5 and 1393) have been the most used. In fact, Torres et al. demonstrated the key role of the implant pores in the anchoring of a bioactive coating. They investigated the coating of diverse c.p. titanium implants with an original bilayer of BGs, 45S5 and 1393, arranged according to their specific characteristics to maximize the osseointegration. In this sense, the 1393 BG layer was in contact with the porous titanium substrate because it presents a theoretically better adherence to the titanium, while the 45S5 BG layer was surrounded by the host bone tissue since it promotes higher bioactivity, demonstrated by solid NMR. The role of the pores was a crucial issue in the anchoring of the coating, both in porosity percentage (30 and 60 vol %) and in pore size range (100−200 and 355−500 μm). The results revealed that the substrate with 30 vol % of porosity and a range of 355−500 μm pore size, coated with this novel BG bilayer, presented the best combination in terms of mechanical and biofunctional properties [ 155 , 158 ]. The bioactivity enhancement induced by the presence of BG was also utilized to prepare biphasic implants required when both bone and cartilage tissues are damaged. In this sense, Torres et al. [ 159 ] described the deposition of BG 45S5-gelatin coatings on porous titanium implants, whereby the BG-polymer composite coating would replace the cartilage and the porous titanium implant would substitute the bone tissue. The preliminary results exhibited a promising route to fabricate composite-coated porous titanium implants as potential candidates to develop alternative treatments for diseases in which tissues of different nature need to be perfectly joined.

Schematic of the different steps that lead to the generation of HA on top of the bioglass particle. Initially, there is an ion exchange on the BG surface that leads to the leaching of calcium and sodium cations from BG, which is replaced by H + from the medium. The acidification of the BG surface entails the liberation of silicic acid, producing a silica-gel interface that absorbs Ca 2+ and phosphate anions. Finally, the amorphous calcium phosphate layer generated crystalizes to HA.
4.2. Organic Coatings
4.2.1. growth factors-based coatings.
Growth factors are a group of molecules involved in the cell division and tissue proliferation. Each growth factor recognizes a specific membrane receptor and their union initiates or inhibits cell division ( Figure 8 ). Main growth factors used in implant coatings are bone morphogenetic proteins (BMPs) and vascular endothelial growth factor (VEGF).

Schematic representation of growth factor activity on protein and enzymes activation, and gene expression in human cells, inducing proliferation: growth factors attach to the receptor, which initiate a signaling cascade. This cascade activates a metabolic that induces enzymes and proteins activation. It also changes gene expression that transcripts and translates to proteins, such as enzymes.
VEGF is a signal protein that has shown to activate gene and protein expression of vasculogenesis and has been found, during in vitro experiments, to enhance primary rat osteoblast proliferation and to increase alkaline phosphatase (ALP) activity. In addition, the in vivo experiment showed an important improvement in the activation of osteoblasts and endothelial cells [ 108 ].
BMPs are proteins with a key role in inducing bone and cartilage formation by the regulation and promotion of osteogenic and bone mesenchymal stem cells. These characteristics have led BMPs to be increasingly applied in dental coatings. In fact, the high demand of these molecules has led to the use of recombinant techniques to obtain them in a good yield. In 2007, the Food and Drug Administration (FDA) approved the recombinant human BMPs (rhBMPs) for therapeutic uses in dentistry, including rhBMP2 and rhBMP7 [ 108 ]. Currently, rhBMP2 is commercially available and has been used for bone regeneration in dental implantology. Although its delivery in the protein form has been tested, best results were obtained when rhBMP2 gene delivery was investigated. In this sense, dosage issues were found in the first case and safe and effective dosage have not been determined yet. Meanwhile, rhBMP2 gene delivery displayed a synthesis of the protein for weeks to months during in vivo experiments, depending on the vector [ 160 ]. However, the use of viral vectors is an important concern when translating these techniques to the clinical practice, although some trials in dentistry have been ongoing. When applied soaked in a poly(ethyl acrylate) coated titanium surface, it showed excellent osseointegration when included in very low concentrations [ 161 ].
A study regarding the effect of anodized implants coated with combined rhBMP2 and recombinant human VEGFs on vertical bone regeneration in the marginal portion of the peri-implant showed alveolar bone regeneration and enhanced bone-implant contact in the microthread [ 162 ].
4.2.2. Extracellular Matrix Proteins and Polisaccarides
The accumulation of extracellular matrix (ECM) proteins is another approach tested to enhance the biocompatibility on implant surfaces. During the growth phase of bone integration, fibroblast growth factor stimulates fibroblasts to secrete ECM proteins, including elastin, chondroitin sulfate collagen, fibronectin, hyaluronic acid, and other proteoglycans [ 108 ]. As examples, Kellesarian et al. [ 163 ] demonstrated that the application of collagen-chondroitin sulfate as an implant coating clearly increased osseointegration by promoting bone formation in the implant–bone interface. The use of mussel adhesive protein was tested by Yin et al. [ 164 ]. These proteins enhance osseointegration promoting the differentiation of bone-forming cells as well as favoring cell adhesion and proliferation. Regarding the improvement of the mechanical properties caused by a rapid osseointegration, Raphael et al. [ 165 ] demonstrated that the use of elastin-like protein for coating implants in a rat tibia and femur led a reduction in the implant micromovements related to a deficient force load. Moreover, the experiments carried out by Sabino et al. [ 166 ] showed that the inclusion of hyaluronic acid on polyelectrolyte multilayer coatings improved osteogenic differentiation of adipose-derived stem cells and bone mineral deposition.
4.3. Antibacterial Strategies of Coatings
Dental-implant failure has been commonly associated with the presence of infections as postoperative complication of restoration. As mentioned in the introduction of this review, bacteria colonization coexists with biofilm, which protects microorganisms on the implant surface. Gram-positive bacteria, (i.e., Staphylococcus epidermidis , Staphylococcus aureus , and Enterococcus spp.) and Gram-negative bacteria, such as Pseudomonas aeruginosa , represent the most common causes related to dental implants infections. In order to develop effective infection-control actions, researchers have explored new coatings that interfered with the two staged mechanism of bacterial adhesion, inhibiting biofilm formation. In fact, when a biofilm layer is formed, the eradication of the infection can be very difficult due to the presence of the exopolysaccharidic matrix, which reduces both the penetration of antibiotics and the vascularization of the site ( Figure 9 ). Thus, the prevention of biofilm formation by selecting antibacterial surfaces, with repelling or killing activity ( Figure 9 ), has been considered a key point to obtain a high rate of implant success during a long period of time after the implantation. Depending on its treatment, implants lead to biofilm formation on their surfaces if these are naked, due to the high biocompatibility of the materials commonly used to fabricate them. However, they could be treated to kill bacteria by a biocidal agent which can be chemically linked to the surface or sustainedly released from it. In addition, antibiofouling treatments could be applied to avoid bacterial adhesion to surfaces, for example, causing steric or electrostatic repulsions or low surface energy by modifying surface topography. Researchers have tried to produce systems for a correct release of drugs and avoiding their excessive application, which induce the growth of drug-resistant bacteria [ 108 ].

Bacterial interactions with implant’s surfaces: ( a ) on naked surface ( b ) on surface with killing activity ( c ) on surface with repelling activity.
In this section, different novel dental implants coatings with antibacterial effect are described in detail, such as drug-releasing systems, antimicrobial surfaces with no-drug release and antifouling biomaterial strategies.
4.3.1. Drug-Releasing Coatings
Taking into account all these facts, the development of original coatings able to release drugs from the implant into the host oral cavity has been considered a therapeutic challenge for researchers over recent decades [ 167 , 168 , 169 ]. During the delivery process, the active molecules can be transferred from the biomaterial to the environment with a controlled approach [ 170 ]. In fact, the coatings of the implant or its bulk structure can allow drugs to be eluted to the environment by diffusion, osmotic pressure, and via matrix degradation for a period of time [ 171 ].
In particular, it has been reported that molecules are released from implants through four main mechanisms, namely diffusion-controlled, solvent-controlled (i.e., osmotic or swelling phenomenon), chemically controlled (i.e., polymer biodegradation and bond cleavage of the drug-biomaterials association) and pH-sensitive mechanisms [ 170 ]. In addition, dissolution, diffusion, portioning, erosion and molecular interactions have been explored as other possible mechanisms for drug release [ 172 , 173 , 174 ]. Enzymatically degradable coatings and soluble biopolymers have been also developed with this aim [ 175 , 176 ]. A proposal of classification has been provided by Stewart et al. [ 177 ], and drug-delivery devices were grouped into two main categories, of passive implants and active implants. Passive systems were considered biodegradable or non-biodegradable, and the active implants subgrouping relied on the type of energy/method driving to release the drug. Once the passive implant is installed, the delivery is governed only by the chemical-physical features of the material, fabrication method and drug formulation. Conversely, in the active release, the whole process is controlled by mechanical, electrical, magnetic, laser or other mechanisms. In addition, since commonly metallic devices have been employed in clinical implantology, researchers have suggested that the trigger for the drug release should be based on external stimuli, providing the required dosage for the healing effect [ 178 ].
The elution time of the active molecule is also affected by surface topographies of the implants and the drug load. Biomaterials such as polymeric matrices, hydrogels and nanoporous matrices have been evaluated for this purpose [ 179 , 180 , 181 , 182 , 183 , 184 , 185 ]. Types of carrier-based drug delivery biomaterials have included dendrimers and micro- and/or nanoparticles (e.g., micro- and nanospheres, nanofibers and nanocapsules) [ 186 ]. In addition, due to the increase in technological advancement, ceramic substrates have also been used as systems for controlled drug release. In fact, a nanometer scale led to the development of mesoporous silicas devices for better control over molecule loading and release kinetics [ 187 ].
The desired properties of an implant-coating drug delivery system include a biocompatible material without secondary side effects both locally and systemically, minimizing complications related to the mismanagement of a drug delivery dose but with high bioactivity to promote osseotintegration. Various modifications of implant healing coatings for in situ infections have been explored by incorporating antibiotics, antimicrobial peptides, and antiseptics [ 109 ]. Additionally, antibiotics have been loaded on the implant surface to destroy pathogens, preventing acute or chronic infections, but avoiding repercussions for eukaryotic cells. Dipping or spray-coating methods have been investigated for the incorporation of antibiotics to the implant with the aim of obtaining a therapeutic drug release coating in order to replace the use of systematic agents, reducing toxic effects [ 188 , 189 , 190 , 191 ]. Gentamycin belongs to broad-spectrum antibiotics, and it has been widely applied in dental implantology for the development of therapeutic coatings with HA [ 192 , 193 ]. A biodegradable gentamicin-polylactic acid-coated implant was studied in rats. It was demonstrated that the addition of 10% gentamycin to the polylactic acid surface adds favorable activity against implant-related infections [ 194 ]. In addition, another in vitro study with nanotubes, made of titanium throughout anodization of the bulk material and loaded with gentamycin, were capable of promoting osteoblastic activity, and had great effects against bacterial adhesion [ 195 ]. Likewise, tetracycline-loaded chitosan coatings have exhibited an improvement of both the bond and retention of blood clots on the host bone, promoting osseointegration [ 196 ]. Other authors evaluated gentamicin-based coating outcomes by employing antibiotic-loaded fibers obtained by electrospinning. They demonstrated an optimum activity for eradicating peri-implantitis-associated bacteria and biofilm development [ 167 ]. Another work confirmed the promotion of early bone healing with tetracycline-incorporated polymer nanofibers by observing that the expressed ALP kinase was relatively higher than for other systems [ 197 ]. However, tobramycin has been loaded instead of gentamicin on implant surfaces, reducing ototoxic effects, and local distribution to the skull at high doses [ 198 ].
A reduced adherence of Staphylococcus aureus to the titanium implant was obtained by the slow release of the agent from a coating formed of vancomycin loaded silica sol–gel film [ 199 ]. Moreover, to formulate vancomycin-loaded coatings with long-lasting anti-adherence activity, the drug was covalently attached to different implant surfaces. Results showed that released vancomycin had the same activity as the standard drug against Staphylococcus aureus , independently of surface morphology. This last aspect indicated that the topography can be tailored to improve the cellular response or osseointegration, with no effect on the total peptide released and positive therapeutic outcomes [ 200 ]. Synergic effects were obtained when researchers loaded polymers such as chitosan and hyaluronic acid with vancomycin-encapsulated titanium nanotubes. These polymers were functionalized with catechol, reducing the concentration of reactive oxygen species, and consequently inflammation, but supporting osseointegration. In addition, it was reported that bacterial hyaluronidase was able to digest hyaluronic acid, producing a slow release of the antimicrobial peptide, which inhibited Staphylococcus aureus attachment in vitro, and promoted the bone healing in an in vivo experiment [ 2 ]. Besides, Cometa et al. took advantage of hydrogels as versatile candidates for drug controlled release by developing vancomycin and ceftriaxone-modified poly(ethylene glycol diacrylate) hydrogel coatings for titanium dental implants. These coatings displayed remarkable antibacterial activity against methicillin-resistant Staphylococcus aureus [ 198 ].
Doxycycline has been commonly employed for the formulation of antibiotic-loaded coating since it suppresses bacteria growth and prevents both peri-implant inflammation and bone resorption. In vivo studies showed that HA coatings treated with doxycycline reduced the progression of peri-implantitis [ 201 ]. Moreover, dental implants formed of titanium nanotube surface coated with polylactic- co -glycolic acid and doxycycline have shown to provide pH-sensitive systems for a controlled release of the drug [ 202 ]. Porphyromonas gingivalis growth has been demonstrated to be inhibited, during a 28 day period interval, by nanotubes loaded with doxycycline on the dental implant surface [ 203 ]. In addition, doxycycline-based coatings were found to prevent microorganism colonization and consequently control of peri-implant mucositis and peri-implantitis [ 204 ].
Nanospray drying technology was used for preparing tailor-made biocompatible nanocoatings of polylactic-co-glycolic acid with antibacterial activity due to the presence of norfloxacin. The technology was applied on titanium discs as material for dental implants, tested in vitro, and showed great activity against bacterial accumulation (i.e., 99.83% of reduction in the number of viable colonies) [ 191 ]. Bacitracin has been covalently fixed to dopamine coatings, and presented strong activity against macrophage spreading, Staphylococcus aureus and its methicillin-resistant counterparts, but promoted adhesion, proliferation, and osteogenic differentiation of the human bone marrow mesenchymal stem cells [ 205 ].
Titanium surfaces have also been functionalized with quercetin, demonstrating that flavonoids promote osteogenic activity. In fact, they increase alkaline phosphatase function and promote mineralization of primary human mesenchymal stem cells, but prevent peri-implantitis since they decrease the release of the inflammatory mediator PGE2 [ 206 ]. Likewise, TiO 2 nanotubes have been loaded with propolis, which contain high levels of flavonoids, and tested in rat mandibles for dental-implant applications. Results confirmed that this type of coating improves cell proliferation and differentiation within a proper osseointegration [ 207 ].
Moreover, chlorhexidine has been considered a broad-spectrum antimicrobial and antifungal agent, and it has been used for the treatment of odontogenic infections. It showed satisfactory antibacterial results when the drug was loaded into microporous silica coatings by diffusion, which avoided the burst release of the drug [ 190 ]. In another experiment, titanium surfaces were coated with chitosan containing 20% tetracycline or 0.02% chlorhexidine digluconate. The results reported that the coatings released 89% of the tetracycline in one week but 100% of chlorhexidine in two days. In addition, released tetracycline inhibited bacterial growth with no cytotoxicity to host cells, and the chlorhexidine presented activity against microorganisms at up to two days, but it was toxic to humans since the first day [ 196 ].
It has been reported that halogenated furanones, extracted from the red alga Delisea pulchra , have strong effects against various pathogens. The unique activity is based on their structure, such as bacterial N -acyl homoserine lactone, which renders halogenated furanones less able to induce drug resistance. In fact, N -acyl homoserine lactone acts as signal molecule in bacterial communication to control population growing and biofilm formation. A novel coating formed of nanoparticles containing a halogenated furanone compound was fabricated for the prevention of peri-implantitis issues. The study concluded with good results on the preventive effect at the early stage [ 208 ].
A slow release of minocycline from microspheres was recently reported during an in vivo experiment. Chitosan-coated alginate and poly(meth)acrylate-glycerin were explored as local minocycline microsphere carriers for the treatment of peri-implant mucositis. Chitosan-based microspheres showed longer effect as carrier, and better bacteriostatic activity than the poly(meth)acrylate-glycerin ones. Nevertheless, authors claimed that the longer drug sustainability did not lead to improved treatment [ 209 ].
4.3.2. “No-Releasing” Coatings
The development of biopolymers with antibacterial effects has represented a feasible option in the field of implants coatings over the last years. In fact, their ability to act as biocides, while avoiding the release of substances, prevents the toxicity arising from the diffusion of the low molecular weight drugs through the polymeric matrix. In addition, these polymers usually present longer-term activity. Thus, biopolymers, due to the intrinsic features, have been commonly used in implants coatings to mitigate, combat and/or eradicate infections, inhibiting bacteria adhesion and biofilm formation [ 210 ]. Francolini et al. gave a definition for these family of coatings: “antimicrobial polymers are usually referred to as polymeric biocides, if obtained from polymerization of bioactive repeating units, or biocidal polymers, when the whole macromolecule is bioactive” [ 198 ]. However, even if the polymerization of active substances induces a decrease in toxicity, it also reduces the antimicrobial effect. It has been reported that vancomycin-modified poly(ethylene glycol methacrylate) resulted in a polymer that was sixfold less effective than the low molecular weight drug [ 211 ]. On the other hand, biocidal polymers present many advantageous properties such as being non-volatile, chemically stable and unable to pass through host skin [ 212 ].
Polycationic polymers are commonly employed since they can establish bonds with the membrane of microbial cells. The mechanism can be briefly described as follows: after the absorption, the polymer penetrates into the cell wall and interacts with lipid or protein of the membrane, thereby inducing disassembly which results in leakage of intracellular small molecules, degradation of proteins and nucleic acids, and eventually cell wall lysis [ 213 ]. In this regard, chitosan and carboxymethyl chitosan, which are polysaccharide of natural origin, formed of N-acetylglucosamine and D-glucosamine that vary in composition, sequence, and molecular chain length, have been the most extensive antimicrobial polymers explored among natural cationic polymers. They are considered agents with a broad spectrum of activity due to their killing effect against Gram-positive and Gram-negative bacteria [ 214 ]. However, they have presented some drawbacks due to unspecific inhibition of osteoblasts and bacteria fixation. Thus, they have been functionalized with agents with adhesion activity such as HA [ 215 ], bone morphogenetic protein-2 [ 216 ], ALP [ 217 ], silica–chitosan hybrid materials [ 218 ], and chitosan-58S BG nanocomposite [ 219 ]. Moreover, it has been demonstrated that the covalent grafting of chitosan to substrates of titanium through a glutaraldehyde linker were more effective than chitosan used in a solution as a killing agent [ 220 ], and in the prevention of biofilm formation by Pseudomonas aeruginosa and Staphylococcus aureus [ 221 ].
Silk is considered a protein polymer and it has been explored as a biomaterial for dental implants. It has been studied for tissue regeneration, alone or in a composite materials (e.g., silk-HA composites). Tissue engineering and regenerative medicine have demonstrated the biocompatibility and low immunogenicity of this natural polymer, so it has been considered a possible strategy for coating applications [ 64 , 222 ]. In addition, antibacterial feature can be included in silk-based coatings, as in the case of silk nanocomposites containing silver nanoparticles [ 223 ]. The analysis of the blend showed the presence of nanoTD particles dispersed in the SF blend, which promoted the capacity to control bacterial growth when compared to pure SF membrane [ 224 ].
Photoactive coatings, based on metal oxide nanoparticles, have represented a second strategy of non-releasing surfaces with antibacterial effect. These systems have demonstrated to generate reactive oxygen species (ROS) in the presence of ultraviolet or visible radiation. ROS damage carbohydrates, lipids, proteins, and DNA, and induce bacterial cell death. Furthermore, TiO 2 , CuO and ZnO nanoparticles showed antibacterial effect due to their ability to promote the presence of oxidative stress linked to photogenerated ROS [ 225 ]. In addition, since nanosystems provide much more surface area than bulk materials, they are more exposed to the radiation with the consequence of producing more ROS. Additionally, TiO 2 represents the most used material employed in this field. In fact, titania was found to promote osteoblast adhesion and proliferation [ 226 ]. Moreover, TiO 2 coatings were able to suppress adhesion of Streptococcus mutans and killing effect against Streptococcus mutans and Porphyromonas gingivalis [ 227 ].
4.3.3. Antifouling Coatings
Antifouling coatings have been gaining momentum in dental implantology due to their great activity for repelling microorganisms. Hydrophilic polymers, zwitterionic materials, and superhydrophobic materials have been employed for this purpose.
Poly(ethylene glycol) (PEG) has been the most explored hydrophilic polymer to obtain coatings with antifouling properties. In fact, PEG chains act against protein adsorption through a steric-repulsion mechanisms, and also due to the formation of a barrier made of structured water associated with PEG [ 228 ]. This component mechanism was investigated for the first time by Jeon et al. in the early 1990s, when it was demonstrated that the interaction between the surface and proteins was inhibited by the repulsive electric forces arising from the compression of the highly mobile PEG, which required the removal of water from the hydrated polymer. Besides, the presence of this tightly bound water layer interacting with the PEG was considered a physical obstacle for protein and pathogens [ 229 ]. Several strategies were used to immobilize PEG on the biomaterials surface, namely self-assembly, physisorption, silanization, electropolymerization, plasma polymerization and pulsed electrodeposition [ 228 , 230 ].
The use of long polymer chains favored more efficient surface coverage, arising from PEG coatings formed by self-assembly. When the process of self-assembly took place on a surface, highly ordered low-dimensional structures can be spontaneously formed onto the surface, the so-called self-assembled monolayers (SAMs) [ 198 ]. In fact, the self-assembly process has been commonly considered as the gold standard to obtain tailored/ordered structures in other fields for clinical and toxicological purposes [ 231 ], and it was defined by Accioni et al. as “the phenomenon by which isolated components organize autonomously and spontaneously into ordered and/or functional structures” [ 232 ]. Moreover, self-assembly provided short PEG chains with protein-resistant activity in the work of Prime and Whitesides, who developed SAMs formed of alkanethiolates displaying short PEG chains [ 233 ]. Recently, SAMs for dental implantology have been considered an easy and precise way to tailor surface properties since these systems are formed of well-organized organic structures with the unique feature of modifying chemical properties of the interface at the molecular scale. Besides, in order to develop new biomaterials, these properties can be tailored during synthesis for obtaining feasible study systems for the interaction between surfaces, proteins, and cells [ 234 ].
The stability of PEG coatings was evaluated and showed good results under sterilization processes and exposure to physiological conditions in phosphate-buffered saline (PBS) [ 198 ]. Controversially, PEG presented some limitations due to the rapid auto-oxidation [ 235 ] when exposed to oxygen, metal ions and some enzymes, which can affect the long-term durability of the coating [ 236 ].
Zwitterionic materials represent the novel generation of polymers with antifouling properties over the last years. They were prepared with biocompatible biomaterials for the development of coatings, resins, adhesives, cements, composites, varnishes, and sealants [ 237 , 238 ]. Their structure results in an overall neutral system because of the presence of an equal number of positively and negatively charged functional groups. In addition, zwitterionic materials have been considered to simulate the biologic membrane of phospholipids, because they are able to arrange themselves in a bilayer with the hydrophilic groups positioned outwards, and the hydrophobic chains positioned inwards. Moreover, they have also been used as super hydrophilic protein-repellent polymers due to the presence of hydrogen-bond acceptor groups rather than donors, which induce a hydration shell trough electrostatic interaction. In this way, fluids with a high protein content, such as saliva, are repelled and biofilm formation can be prevented [ 239 ].
Coating of a surface with the zwitterionic 2-methacryloyloxyethyl phosphorylcholine (MPC) co-polymer has been demonstrated to reduce the retention of the pathogens Staphylococcus aureus , Streptococcus mutans , Pseudomonas aeruginosa , and Candida albicans , attributed to the superhydrophilicity of MPC-coated. Other researchers reported that MPC coatings decrease the adhesion on plastic coverslips and HA disks by common microorganisms and peri-implant pathogens. In addition, MPC have been combined with quaternary ammonium compounds (QACs) to obtain a synergic clinical effect. It was demonstrated that the contact-killing activity of QACs can be reduced in presence of salivary pellicle, which is reduced by the protein-repellent effect of MPC [ 240 ]. However, in previous studies, MPC-based coatings compromised the mechanical properties of the bulk dental material. Thus, some authors reported sufficient results when MPC was photochemically added onto the surface of filled composite resins during the dental procedure [ 241 ]. A new polymer made of MPC, n -butyl methacrylate and 2-metahcryloyloxyethyl-4-azidobenzoate was explored as an antifouling coating. It showed great ability to inhibit both the formation of plaque on the coated implant, and to withstand chemical and mechanical stressors until to two weeks. Moreover, it also demonstrated a reduction in Fusobacterium nucleatum and Streptococci spp. during 5 h in a crossover trial [ 242 ].
Superhydrophobic materials have been explored as coating (contact angle greater than 150°) for dental implants because they showed great antibiofilm properties. A superhydrophobic mechanism was reported to be based on the change of the wettability on the implant surface, reducing adhesion of molecules and cells to the coating through charge or hydrophobic interactions [ 243 , 244 ]. Various techniques have been adopted to produce superhydrophobic materials, including chemical vapor deposition, fluorine-based polymer, and laser system among the others two steps strategies. On the other hand, other authors developed a one-step methodology to fabricate a superhydrophobic coating for the titanium implants using glow discharge plasma. The results demonstrated an improvement in corrosion resistance, good biocompatibility, antimicrobial ability, and a reduction of colonization by oral pathogens [ 245 ].
5. Latest Trends in Analytical Chemistry
The therapeutic impact of dental implants on human health has led to the need to develop reliable analytical methods as pillar for the evaluation of new biomaterial-based devices. Moreover, Singh et al. reported that “oral fluids (saliva) contains the biomarker and serum proteome components which enables the identification of both oral and systemic diseases” [ 246 ]. The quantification of markers’ concentration have had a very important role, due to the limitations of common methods for diagnosis and prognosis of peri-implantitis and oral issues [ 232 , 247 , 248 ]. In addition, the pharmacokinetics and pharmacodynamics properties of drug release from a biomaterial-based implant can be evaluated throughout high performance liquid chromatography coupled with mass spectrometry ( Figure 10 ) or with an ultraviolet apparatus, considered the gold standard in this field [ 197 , 249 , 250 , 251 ]. In is reported, in the United States of Pharmacopeial Convention, that “the types of chromatography useful in qualitative and quantitative analysis employed in USP procedure are column, gas, paper, thin-layer (including high-performance thin-layer chromatography), and pressurized liquid chromatography (commonly called high-pressure or high performance liquid chromatography)”, where USP stands for United States Pharmacopeia [ 252 ].

Schematic drawing of sample analysis by the use of a mass spectrometer. Briefly, the instrument consists of three components: ( a ) ion source, where gaseous ions are formed; ( b ) detector system, for detecting the ions. ( c ) Analyzer, for resolving the ions in accordance with their mass-to-charge ratio (m/z). The multiple ions generated from the injected sample are separated in function of m/z, and then recorded by considering their relative abundance.
The determination of some antibiotics has been extensively investigated. Thus, a gentamycin release profile has been carried out via high-performance liquid chromatography (HPLC), equipped with a reverse phase column, and coupled to an ion trap mass spectrometer with an electrospray ionizer. A mass spectrometry (MS) analysis confirmed the presence of three ions for the three components that form gentamycin (i.e., gentamicyn C1a, C2, and C1). The sum of these three most abundant ions was used for the calculation of gentamicin concentration [ 253 ]. Tobramycin quantification has represented a challenge in analytical chemistry due to its physico-chemical properties (i.e., basicity, hydrophilicity and lack of a UV absorbing chromophore) [ 254 ]. Sorensen et al. [ 112 ] evaluated the level of tobramycin incorporated and released from pins in PBS at 37 °C and analyzed by HPLC according to the British Pharmacopoeia and Fabre et al. [ 255 ], by the use of precolumn derivatization of the aminoglycoside antibiotic. On the other hand, tetracycline concentration released by nanofibers for dental coatings was easily determined by HPLC equipped with UV-Vis detector [ 167 ]. Moreover, in this last section, a brief panorama of novel analytical trends related to the use of biomaterials in dental implantology is presented, including the latest concept on the correlation between the etiogenesis of peri-implantitis and the degradation of the implant surface.
5.1. OMICS in Dental Implantology
Mass spectrometry has been considered the most comprehensive strategy in “OMICS” [ 256 ], which refers to scientific fields ending with –omics such as genomics, transcriptomics, proteomics, or metabolomics [ 257 ]. Besides, the development of analytical methods to characterize polymer sequences has represented a challenge in current polymer science, due to efforts to tailor the properties of biomaterials for medical purposes, among others [ 256 ]. In addition, OMICS technologies have gained momentum in uncovering molecules and signaling pathways related to bone formation and osseointegration, in order to provide personalized treatment in dentistry and implantology [ 258 ]. The bioconjugate BMP2-(PEO-HA)2, composed of a dendron with two monodisperse poly(ethylene oxide) branches functionalized with a HA binding peptide, and a focal point substituted with a bone-growth stimulating peptide (BMP2), was successfully characterized by MS methods. With this aim, the authors took advantages of various techniques including matrix-assisted laser desorption ionization (MALDI), electrospray ionization (ESI), tandem mass spectrometry (MS/MS), and ion mobility mass spectrometry (IM-MS) [ 259 ].
5.2. Detemination of Biomarkers for the Evaluation of Biomaterial Effect and Clinic Outcomes
The National Institutes of Health Biomarkers Definitions Working Group defined biomarkers as “a characteristic that is objectively measured and evaluated as an indicator of normal biological processes, pathogenic processes, or pharmacologic responses to a therapeutic intervention” [ 260 ]. The proper evaluation of biomarkers in an oral cavity can represent a useful tool for evaluating the activity-therapeutic effect of new biomaterial used for dental-implant coating.
It was reported that various biomarkers were explored for the assessment of bone regeneration and healing around biomaterials. Many biomaterials were tested (e.g., titanium dental implants with surface modifications, and scaffolds loaded with drugs, osteogenic cells, or biological factors, etc.) in different trials. Bone regeneration, resulting in a biomarkers increase was reported in all these studies [ 261 ]. In particular, the rate of success of titanium dental implants can be monitored by the assessment of markers of osseointegration such as OCN (osteocalcin) and COL-1 (collagen type I) in the post-implant placement period [ 262 , 263 , 264 ]. In another study where the titanium dental implant was coated with a fluoride-material, the expression of OCN, RUNX-2 (Runt-related transcription factor 2), and COL-1 was associated with the positive effect of fluoride upon bone formation [ 265 ]. Likewise, a simvastatin coating proposed for in vivo trial, demonstrated the promotion of angiogenesis and osseointegration with the increased expression of VEGF and ALP markers [ 261 , 264 ].
With a similar aim, Kumar et al. evaluated the response of peri-implant connective tissue to titanium and zirconia abutments via the evaluation of MMP8 assayed by ELISA [ 266 ]. In fact, during the last decade the number of studies examining biomarkers in oral fluids as diagnostic tool for periodontal disease has represented a new trend. Since the 1920s, there have been many changes in the classification of periodontal diseases in order to obtain a proper diagnosis for the further treatment [ 8 ]. Saliva, gingival crevicular fluid, peri-implant sulcular fluid, and mouth rinse remnant have been considered non-invasive and biomarkers and high-content sources to reflect periodontal health. Furthermore, MMP8 (matrix metalloproteinase), MMP9, MMP13, MMP14, IL1β (interleukin), IL10, TIMP1 (metallopeptidase inhibitor), elastase, cathepsin G, cathepsin B, trypsin-like enzyme, sialidase, VEGF, RANKL (receptor activator of nuclear factor kappa beta), OPG (osteoprotegerin), TGF-β1 (tumor growth factor), nicotine, cotinine, cystatin C, MPO (myeloperoxidase), PAF (platelet-activating factor), and lactoferrin represent the common markers that researchers assayed over the last decade. On the other hand, ELISA, immunofluorometric assay (IFMA), colorimetric assay and chromatography-tandem MS have been selected for their determination, as previously reported by Gul et al. [ 247 ].
5.3. Analytical Methods for the Characterization of Micro- and Nanosized Implant-Related Particles and Metals in Inflamed Peri-Implants Ttissues
An emerging concept about etiopathogenesis of peri-implantitis has explored the degradation of the implant surface with the consequent particle release as an inflammation catalyst mechanism [ 267 ]. This phenomenon has been deeply studied for orthopedic implants, whereby the deterioration of metal biomaterials was demonstrated to accumulate exogenous particles in the peri-implant milieu [ 268 ]. Likewise, some authors reported that in dental devices, damage to the surface can be caused by both surface instrumentation and dynamic interactions in the implant–abutment interface; however, for titanium devices this can happen, independently from wear corrosion, following a no pre-clinical studied pathway [ 267 , 269 ]. In addition, differences among cell compositions were noticed in case of inflammation in peri-implantitis and periodontitis, with higher macrophage polarization in the first case [ 270 , 271 ]. However, other histological studies, conducted in presence of inflammation around titanium and ceramic implants, demonstrated that implantitis issues depend on a patient-level rather than a material level [ 272 ].
Taking into account that implant-surface degradation may result in the release of titanium ions, as well as particles, which leads to peri-implant inflammation and clinical failure, and it was hypothesized that release can occur in cases for which titanium implants are exposed to corrosion. This study demonstrated, for the first time, via synchrotron X-ray fluorescence mapping, a scattered and heterogeneous distribution of titanium in inflamed tissues [ 273 ]. Besides, the release of particles from Ti and ZrO 2 implants has been observed in pigs after 12 weeks of implant placement [ 274 ]. Thus, to explore size, distribution, and the chemical speciation of substances that can be released from dental implants, Nelson et al. proposed a synchrotron-based characterization of micro- and nanosized implant-related particles arising from Ti and ZrO 2 dental implants in patients with peri-implantitis. For this purpose, synchrotron μ-XRF, nano-XRF and μ-XANES were employed as an analytical tool. They also tried to explain the mechanism behind particle release from ceramic implants as a consequence of stress that induces the local transformation of zirconia from a tetragonal to a monoclinic crystalline phase, susceptible to microlesions. The study concluded by reporting on the presence of Ti particles, with variable speciation, in all tissue sections implanted with Ti devices, as well as ceramic products were identified in five out of eight tissue samples around ceramic implants [ 267 ].
Because of the possibility of degradation of the implant surface within metals release, high performance liquid chromatography coupled with inductively coupled plasma mass spectrometry (HPLC-ICP-MS) was used for the study of distribution and chemical speciation of metals [ 275 , 276 ]. Balcaen et al. developed a reliable method for the determination of trace levels (limit of detection down to 3 ng L −1 ) of titanium in human serum, based on the use of ICP-MS/MS. In fact, it has been reported that the presence of implants in the human body can result in the high presence of titanium in serum [ 277 ]. Other researchers provided HPLC-ICP-MS ( Figure 11 ) as a method for the estimation of total chromium and Cr(III) and Cr(VI) species released from metal implants into whole blood and joint effusion. The results showed higher chromium levels in joint effusion samples obtained from implanted patients [ 275 ].

The illustration represents an example of sample analysis carried out by HPLC coupled to inductively coupled plasma mass spectrometry to measure elements at trace levels in samples. Briefly, the instrument consists of the following components: ( a ) HPLC system, where the chromatographic separation of the sample takes place; ( b ) ICP system, where plasma is ionized by inductively heating the gas (i.e., Argon) with an electromagnetic coil; it represents the ionization source. ( c ) MS detector system, for detecting the formed ions.
Laser ablation-inductively-coupled-plasma mass spectrometry (LA-ICP-MS) was employed as an analytical procedure for the determination of elements derived from titanium implants and physiological elements in soft tissues. Results showed a quantitative mapping of Ti and Al released from dental implant and Mg, Ca, Fe, Zn, Cu, Mn as physiological elements in oral mucosa. Moreover, authors were able to obtain two-dimensional maps of distribution of elements in tested samples which confirmed the release of Ti and Al derived from implants [ 278 ].
6. Conclusions and Future Perspectives
These results broaden the understanding of the development of new materials within the area of dental implantology, suggesting that new multidisciplinary research is needed to improve the biological, physical, and chemical performance of these prostheses. An examination from an engineering perspective shows that various strategies, including the correct selection of both biomaterial composition and surface/geometry of the implant, confirm that they play a key role in achieving good compatibility and promote osseointegration after implantation surgery. Additionally, the implementation of organic- or inorganic-based coatings to promote not only osseointegration but also efficient pharmacological devices that could act as excellent therapeutic agents, including a polymer matrix that allows smart drug delivery in situ, emphasizes the importance of developing future method by which to cope with the 5–11% of dental implants that fail.
Beyond the prospect of exploring accurate analytical methods to evaluate the efficiency of these devices, MS appears as one of the most adequate, since it covers the molecule composition to unravel the mechanistic pathways of complex reactions such as bone formation. Moreover, clinical studies are being employed to manage the implication of biomarkers related to inflammation, osteointegration, or healing procedures via ELISA to measure and evaluate the activity and therapeutic effect of novel biomaterials, biological processes, and pathogenic responses.
This compilation provides a jumping-off point for the use of new biomaterial design for dental implantology and will bring research attention to include alternative methods for a better and more complete understanding of processes from an interdisciplinary point of view. Furthermore, not only will these advancements pave the way for a brighter future for patients with weak or sparse bone who will probably enjoy a better prognosis for successful implants, but other areas such as nanodentistry or nanotechnology are also expected to impact not only diagnosis, but also materials, to support the idea that better is not enough; the best is yet to come.
Author Contributions
Conceptualization, A.A. and B.B; methodology, B.B., J.V. and M.M.; formal analysis, F.A.; investigation, B.B., J.V. and F.A.; resources, B.B.; data curation, M.M., F.A.; writing—original draft preparation, F.A. and J.V.; writing—review and editing, A.A., B.B., J.V., M.M. and F.A.; supervision, A.A. and B.B.; project administration and funding acquisition, A.A. All authors have read and agreed to the published version of the manuscript.
This work was supported by the Ministry of Science and Innovation of Spain under the grant PID2019-109371GB-I00 and of the Junta de Andalucía (Spain) through the Projects PAIDI 2020, P20_00671 and FEDER Andalucía US-1380878; together with projects PPI505/2020 and PPI532/2020 (Universidad de Sevilla, Spain).
Institutional Review Board Statement
Not applicable.
Informed Consent Statement
Data availability statement, conflicts of interest.
The authors declare no conflict of interest.
Publisher’s Note: MDPI stays neutral with regard to jurisdictional claims in published maps and institutional affiliations.
The patient experience of dental implant surgery: a literature review of pertinent qualitative studies
- Review Article
- Published: 27 July 2020
- Volume 190 , pages 835–842, ( 2021 )
Cite this article
- Sinéad O’ Dwyer ORCID: orcid.org/0000-0003-0135-0865 1 &
- Richeal Ní Riordain 2 , 3
656 Accesses
Explore all metrics
This review aims to identify and summarise the findings of published qualitative studies relating to patients’ experiences of dental implant surgery, by means of textual narrative synthesis. A comprehensive two-stage electronic and manual search of the literature identified relevant qualitative studies up to January 2020. Included primary studies ( n = 15) used qualitative research methods including interviews and focus groups to investigate patients’ experiences of dental implant treatment. They looked at the experience of tooth loss, the decision making process, the pre-implant experience, motivating factors and barriers for treatment and the post-implant experience with the prosthesis. There is a deficiency in the exploration of the patients’ intraoperative dental surgical experience and the adjunctive effects of conscious sedation. The included studies give considerable insight into patients’ experiences of the dental implant journey, which in the main, had overall positive consensus. The limited information available regarding patients’ experience of the intra operative dental implant surgery with or without conscious sedation warrants further investigation. This information is a fundamental step to understanding the patients’ preferences, needs and values and ultimately enhancing the quality of patient care.
This is a preview of subscription content, log in via an institution to check access.
Access this article
Price includes VAT (Russian Federation)
Instant access to the full article PDF.
Rent this article via DeepDyve
Institutional subscriptions
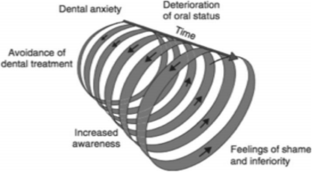
Similar content being viewed by others
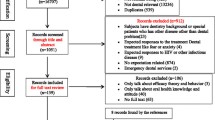
Patients' expectations from dental implants: a systematic review of the literature
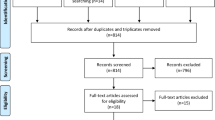
Surgical safety checklists for dental implant surgeries—a scoping review
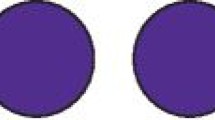
The benefits of implant-retained overdentures as a treatment modality for patients in primary care settings
Health in Ireland: key trends (2018) https://www.gov.ie/en/press-release/-health-in-ireland-key-trends-pdf . Accessed 03/04/2020
Aideen Sheehan CM, Brian O’Connell (2017) Oral health and wellbeing in older Irish adults. TILDA
Hakeberg M, Berggren U, Carlsson SG (1992) Prevalence of dental anxiety in an adult population in a major urban area in Sweden. Community Dent Oral Epidemiol 20(2):97–101. https://doi.org/10.1111/j.1600-0528.1992.tb00686.x
Article CAS PubMed Google Scholar
Carlsson V, Hakeberg M, Blomkvist K, Wide Boman U (2014) Orofacial esthetics and dental anxiety: associations with oral and psychological health. Acta Odontol Scand 72(8):707–713. https://doi.org/10.3109/00016357.2014.898786
Article PubMed Google Scholar
Bernson JM, Elfström ML, Hakeberg M (2013) Dental coping strategies, general anxiety, and depression among adult patients with dental anxiety but with different dental-attendance patterns. Eur J Oral Sci 121(3 Pt 2):270–276. https://doi.org/10.1111/eos.12039
Kutkut A, Bertoli E, Frazer R, Pinto-Sinai G, Fuentealba Hidalgo R, Studts J (2018) A systematic review of studies comparing conventional complete denture and implant retained overdenture. J Prosthodont Res 62(1):1–9
Article Google Scholar
Cronin M, Meaney S, Jepson NJ, Allen PF (2009) A qualitative study of trends in patient preferences for the management of the partially dentate state. Gerodontology 26(2):137–142
Sherwood G (1999) Meta-synthesis: merging qualitative studies to develop nursing knowledge. Int J Hum Caring 3(1):37–42
Programme CAS (2018) CASP checklist: 10 questions to help you make sense of a qualitative research. https://casp-uk.net/casp-tools-checklists . Accessed 02/04/2020
Dixon-Woods M, Shaw RL, Agarwal S, Smith JA (2004) The problem of appraising qualitative research. Qual Saf Health Care 13(3):223–225
Article CAS Google Scholar
Nogueira TE, Dias DR, Rios LF, Silva ALM, Jordão LMR, Leles CR (2019) Perceptions and experiences of patients following treatment with single-implant mandibular overdentures: a qualitative study. Clin Oral Implants Res 30(1):79–89
Kashbour WA, Rousseau N, Thomason JM, Ellis JS (2017) Patients' perceptions of implant placement surgery, the post-surgical healing and the transitional implant prostheses: a qualitative study. Clin Oral Implants Res 28(7):801–808
Abrahamsson KH, Wennstrom JL, Berglundh T, Abrahamsson I (2017) Altered expectations on dental implant therapy; views of patients referred for treatment of peri-implantitis. Clin Oral Implants Res 28(4):437–442
Wang G, Gao X, Lo EC (2015) Public perceptions of dental implants: a qualitative study. J Dent 43(7):798–805
Atieh MA, Morgaine KC, Duncan WJ (2016) A qualitative analysis on participants' perspectives on oral implants. Clin Oral Implants Res 27(3):383–391
Exley C, Rousseau N, Donaldson C, Steele JG (2012) Beyond price: individuals' accounts of deciding to pay for private healthcare treatment in the UK. BMC Health Serv Res 12:53
Rousseau N, Steele J, May C, Exley C (2014) ‘Your whole life is lived through your teeth’: biographical disruption and experiences of tooth loss and replacement. Sociol Health Illn 36 (3):462–476
Grey EB, Harcourt D, O'Sullivan D, Buchanan H, Kilpatrick NM (2013) A qualitative study of patients’ motivations and expectations for dental implants. Br Dent J 214:E1
Lantto A, Wardh I (2013) Dental implants in the functionally impaired: experience from the patients' perspective. Acta Odontol Scand 71(3–4):525–533
Osman RB, Morgaine KC, Duncan W, Swain MV, Ma S (2014) Patients’ perspectives on zirconia and titanium implants with a novel distribution supporting maxillary and mandibular overdentures: a qualitative study. Clin Oral Implants Res 25(5):587–597
Narby B, Hallberg U, Bagewitz IC, Soderfeldt B (2012) Grounded theory on factors involved in the decision-making processes of patients treated with implant therapy. Int J Prosthodont 25(3):270–278
PubMed Google Scholar
Johannsen A, Westergren A, Johannsen G (2012) Dental implants from the patients perspective: transition from tooth loss, through amputation to implants - negative and positive trajectories. J Clin Periodontol 39(7):681–687
Ellis JS, Levine A, Bedos C, Mojon P, Rosberger Z, Feine J, Thomason JM (2011) Refusal of implant supported mandibular overdentures by elderly patients. Gerodontology 28(1):62–68
Hyland R, Ellis J, Thomason M, El-Feky A, Moynihan P (2009) A qualitative study on patient perspectives of how conventional and implant-supported dentures affect eating. J Dent 37(9):718–723
Trulsson U, Engstrand P, Berggren U, Nannmark U, Branemark PI (2002) Edentulousness and oral rehabilitation: experiences from the patients' perspective. Eur J Oral Sci 110(6):417–424
Moher D, Liberati A, Tetzlaff J, Altman DG (2009) Preferred reporting items for systematic reviews and meta-analyses: the PRISMA statement. BMJ 339:25–35
Esposito M, Grusovin M, Shane Chew Y, Coulthard P, Worthington H (2009) One-stage versus two-stage implant placement. A Cochrane systematic review of randomised controlled clinical trials, vol 2
Lalabonova CK (2015) Impact of dental anxiety on the decision to have implant treatment. Folia Med 57(2):116–121
Download references
Author information
Authors and affiliations.
University College Cork, Cork, Ireland
Sinéad O’ Dwyer
Cork University Dental School and Hospital, Wilton, Cork, T12 E8YV, Ireland
Richeal Ní Riordain
University College London Eastman Dental Institute, London, UK
You can also search for this author in PubMed Google Scholar
Contributions
Dr. Sinéad O′ Dwyer had the idea for the review article, performed the literature search and data analysis, and drafted the work. It was then critically revised by Dr. Richeal Ni Riordain.
Corresponding author
Correspondence to Sinéad O’ Dwyer .
Ethics declarations
Conflict of interest.
The authors declare that they have no conflict of interest.
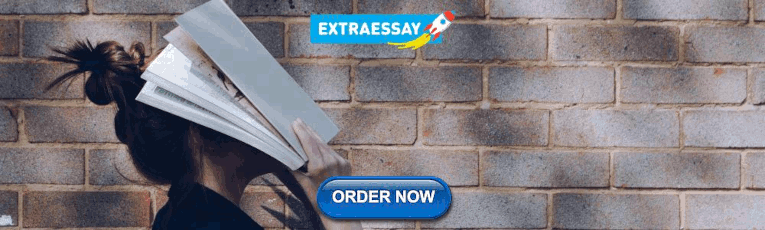
Ethics approval
Not applicable.
Consent to participate
Consent for publication, additional information, publisher’s note.
Springer Nature remains neutral with regard to jurisdictional claims in published maps and institutional affiliations.
Rights and permissions
Reprints and permissions
About this article
O’ Dwyer, S., Riordain, R.N. The patient experience of dental implant surgery: a literature review of pertinent qualitative studies. Ir J Med Sci 190 , 835–842 (2021). https://doi.org/10.1007/s11845-020-02327-y
Download citation
Received : 05 June 2020
Accepted : 23 July 2020
Published : 27 July 2020
Issue Date : May 2021
DOI : https://doi.org/10.1007/s11845-020-02327-y
Share this article
Anyone you share the following link with will be able to read this content:
Sorry, a shareable link is not currently available for this article.
Provided by the Springer Nature SharedIt content-sharing initiative
- Dental implant surgery
- Patients’ experience
- Qualitative research
- Find a journal
- Publish with us
- Track your research
Suggestions or feedback?
MIT News | Massachusetts Institute of Technology
- Machine learning
- Social justice
- Black holes
- Classes and programs
Departments
- Aeronautics and Astronautics
- Brain and Cognitive Sciences
- Architecture
- Political Science
- Mechanical Engineering
Centers, Labs, & Programs
- Abdul Latif Jameel Poverty Action Lab (J-PAL)
- Picower Institute for Learning and Memory
- Lincoln Laboratory
- School of Architecture + Planning
- School of Engineering
- School of Humanities, Arts, and Social Sciences
- Sloan School of Management
- School of Science
- MIT Schwarzman College of Computing
Adhesive coatings can prevent scarring around medical implants
Press contact :, media download.
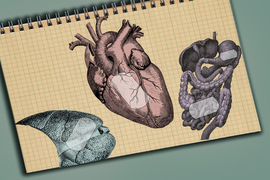
*Terms of Use:
Images for download on the MIT News office website are made available to non-commercial entities, press and the general public under a Creative Commons Attribution Non-Commercial No Derivatives license . You may not alter the images provided, other than to crop them to size. A credit line must be used when reproducing images; if one is not provided below, credit the images to "MIT."
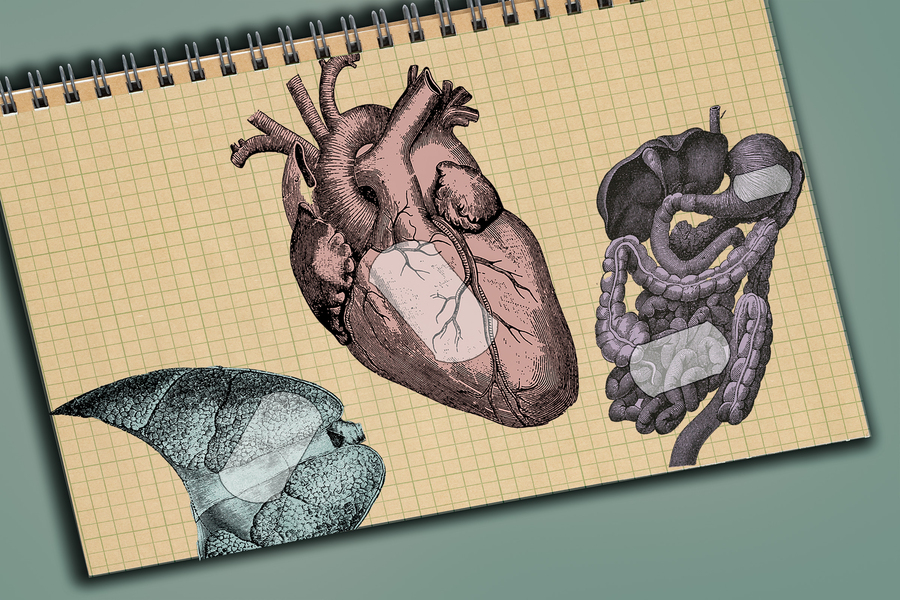
Previous image Next image
When medical devices such as pacemakers are implanted in the body, they usually provoke an immune response that leads to buildup of scar tissue around the implant. This scarring, known as fibrosis, can interfere with the devices’ function and may require them to be removed.
In an advance that could prevent that kind of device failure, MIT engineers have found a simple and general way to eliminate fibrosis by coating devices with a hydrogel adhesive. This adhesive binds the devices to tissue and prevents the immune system from attacking it.
“The dream of many research groups and companies is to implant something into the body that over the long term the body will not see, and the device can provide therapeutic or diagnostic functionality. Now we have such an ‘invisibility cloak,’ and this is very general: There’s no need for a drug, no need for a special polymer,” says Xuanhe Zhao, an MIT professor of mechanical engineering and of civil and environmental engineering.
The adhesive that the researchers used in this study is made from cross-linked polymers called hydrogels, and is similar to a surgical tape they previously developed to help seal internal wounds. Other types of hydrogel adhesives can also protect against fibrosis, the researchers found, and they believe this approach could be used for not only pacemakers but also sensors or devices that deliver drugs or therapeutic cells.
Zhao and Hyunwoo Yuk SM ’16, PhD ’21, a former MIT research scientist who is now the chief technology officer at SanaHeal, are the senior authors of the study, which appears today in Nature . MIT postdoc Jingjing Wu is the lead author of the paper.
Preventing fibrosis
In recent years, Zhao’s lab has developed adhesives for a variety of medical applications, including double-sided and single-sided tapes that could be used to heal surgical incisions or internal injuries. These adhesives work by rapidly absorbing water from wet tissues, using polyacrylic acid, an absorbent material used in diapers. Once the water is cleared, chemical groups called NHS esters embedded in the polyacrylic acid form strong bonds with proteins at the tissue surface. This process takes about five seconds.
Several years ago, Zhao and Yuk began exploring whether this kind of adhesive could also help keep medical implants in place and prevent fibrosis from occurring.
To test this idea, Wu coated polyurethane devices with their adhesive and implanted them on the abdominal wall, colon, stomach, lung, or heart of rats. Weeks later, they removed the device and found that there was no visible scar tissue. Additional tests with other animal models showed the same thing: Wherever the adhesive-coated devices were implanted, fibrosis did not occur, for up to three months.
“This work really has identified a very general strategy, not only for one animal model, one organ, or one application,” Wu says. “Across all of these animal models, we have consistent, reproducible results without any observable fibrotic capsule.”
Using bulk RNA sequencing and fluorescent imaging, the researchers analyzed the animals’ immune response and found that when devices with adhesive coatings were first implanted, immune cells such as neutrophils began to infiltrate the area. However, the attacks quickly quenched out before any scar tissue could form.
“For the adhered devices, there is an acute inflammatory response because it is a foreign material,” Yuk says. “However, very quickly that inflammatory response decayed, and then from that point you do not have this fibrosis formation.”
One application for this adhesive could be coatings for epicardial pacemakers — devices that are placed on the heart to help control the heart rate. The wires that contact the heart often become fibrotic, but the MIT team found that when they implanted adhesive-coated wires in rats, they remained functional for at least three months, with no scar tissue formation.
“The formation of fibrotic tissue at the interface between implanted medical devices and the target tissue is a longstanding problem that routinely causes failure of the device. The demonstration that robust adhesion between the device and the tissue obviates fibrotic tissue formation is an important observation that has many potential applications in the medical device space,” says David Mooney, a professor of bioengineering at Harvard University, who was not involved in the study.
Mechanical cues
The researchers also tested a hydrogel adhesive that includes chitosan, a naturally occurring polysaccharide, and found that this adhesive also eliminated fibrosis in animal studies. However, two commercially available tissue adhesives that they tested did not show this antifibrotic effect because the commercially available adhesives eventually detached from the tissue and allowed the immune system to attack.
In another experiment, the researchers coated implants in hydrogel adhesives but then soaked them in a solution that removed the polymers’ adhesive properties, while keeping their overall chemical structure the same. After being implanted in the body, where they were held in place by sutures, fibrotic scarring occurred. This suggests that there is something about the mechanical interaction between the adhesive and the tissue that prevents the immune system from attacking, the researchers say.
“Previous research in immunology has been focused on chemistry and biochemistry, but mechanics and physics may play equivalent roles, and we should pay attention to those mechanical and physical cues in immunological responses,” says Zhao, who now plans to further investigate how those mechanical cues affect the immune system.
Yuk, Zhao, and others have started a company called SanaHeal, which is now working on further developing tissue adhesives for medical applications.
“As a team, we are interested in reporting this to the community and sparking speculation and imagination as to where this can go,” Yuk says. “There are so many scenarios in which people want to interface with foreign or manmade material in the body, like implantable devices, drug depots, or cell depots.”
The research was funded by the National Institutes of Health and the National Science Foundation.
Share this news article on:
Press mentions, interesting engineering.
MIT engineers have developed a new adhesive, low-cost hydrogel that can stop fibrosis often experienced by people with pacemakers and other medical devices, reports for Maria Bolevich Interesting Engineering . “These findings may offer a promising strategy for long-term anti-fibrotic implant–tissue interfaces,” explains Prof. Xuanhe Zhao.
Previous item Next item
Related Links
- Xuanhe Zhao
- Hyunwoo Yuk
- Jingjing Wu
- Department of Mechanical Engineering
- Department of Civil and Environmental Engineering
Related Topics
- Mechanical engineering
- Civil and environmental engineering
- Biomedical engineering
- Medical devices
- National Institutes of Health (NIH)
- National Science Foundation (NSF)
Related Articles
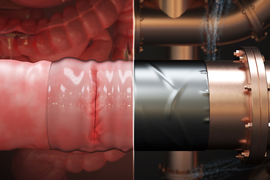
Engineers develop surgical “duct tape” as an alternative to sutures
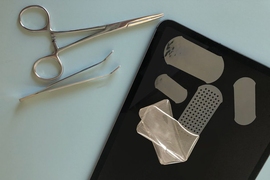
Double-sided tape for tissues could replace surgical sutures
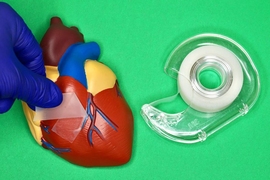
Super-strong surgical tape detaches on demand
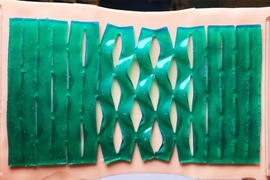
Paper-folding art inspires better bandages
More mit news.
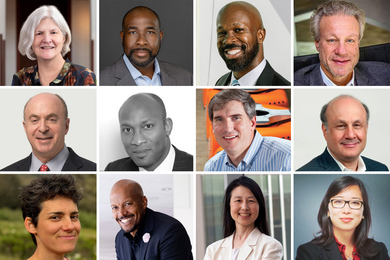
MIT Corporation elects 10 term members, two life members
Read full story →
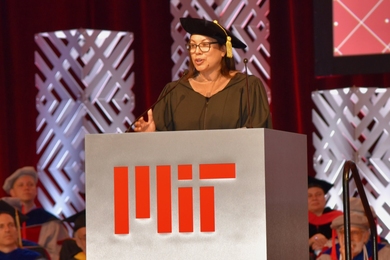
Diane Hoskins ’79: How going off-track can lead new SA+P graduates to become integrators of ideas
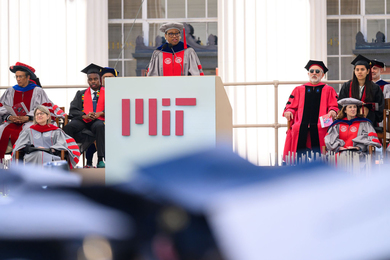
Chancellor Melissa Nobles’ address to MIT’s undergraduate Class of 2024
Noubar Afeyan PhD ’87 gives new MIT graduates a special assignment
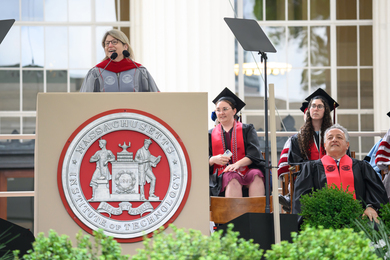
President Sally Kornbluth’s charge to the Class of 2024
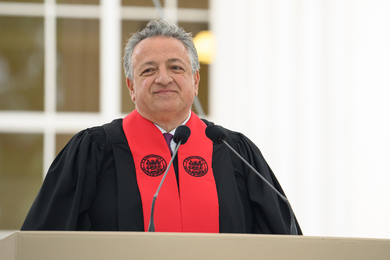
Commencement address by Noubar Afeyan PhD ’87
- More news on MIT News homepage →
Massachusetts Institute of Technology 77 Massachusetts Avenue, Cambridge, MA, USA
- Map (opens in new window)
- Events (opens in new window)
- People (opens in new window)
- Careers (opens in new window)
- Accessibility
- Social Media Hub
- MIT on Facebook
- MIT on YouTube
- MIT on Instagram
Click through the PLOS taxonomy to find articles in your field.
For more information about PLOS Subject Areas, click here .
Loading metrics
Open Access
Peer-reviewed
Research Article
Predictors of cochlear implant outcomes in pediatric auditory neuropathy: A matched case-control study
Roles Conceptualization, Data curation, Formal analysis, Investigation, Methodology, Project administration, Resources, Supervision, Validation, Visualization, Writing – original draft, Writing – review & editing
Affiliations Audiology and Speech-Language Pathology Program, University of Ottawa, Ottawa, Ontario, Canada, School of Communication Sciences and Disorders (SCSD), Dalhousie University, Halifax, NS, Canada
Roles Conceptualization, Methodology, Validation, Writing – review & editing
Affiliations Audiology and Speech-Language Pathology Program, University of Ottawa, Ottawa, Ontario, Canada, Child Hearing Lab, CHEO Research Institute, University of Ottawa, Ottawa, Ontario, Canada

Roles Validation, Writing – review & editing
Affiliations Department of Otolaryngology-Head and Neck Surgery, University of Ottawa, Ottawa, Ontario, Canada, Department of Otolaryngology-Head and Neck Surgery, The Ottawa Hospital, Ottawa, Ontario, Canada
Affiliation Speech and Language Pathology, and Otolaryngology Department, Necker Hospital, Paris, France
Roles Conceptualization, Data curation, Formal analysis, Investigation, Methodology, Project administration, Resources, Supervision, Validation, Visualization, Writing – review & editing
* E-mail: [email protected]
Affiliation Audiology and Speech-Language Pathology Program, University of Ottawa, Ottawa, Ontario, Canada
- Zahra Jafari,
- Elizabeth M. Fitzpatrick,
- David R. Schramm,
- Isabelle Rouillon,
- Amineh Koravand
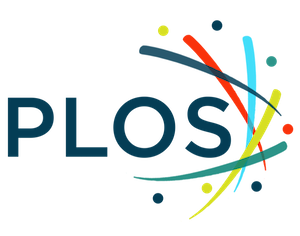
- Published: May 29, 2024
- https://doi.org/10.1371/journal.pone.0304316
- Reader Comments
Current evidence supports the benefits of cochlear implants (CIs) in children with hearing loss, including those with auditory neuropathy spectrum disorder (ANSD). However, there is limited evidence regarding factors that hold predictive value for intervention outcomes.
This retrospective case-control study consisted of 66 children with CIs, including 22 with ANSD and 44 with sensorineural hearing loss (SNHL) matched on sex, age, age at CI activation, and the length of follow-up with CIs (1:2 ratio). The case and control groups were compared in the results of five open-set speech perception tests, and a Forward Linear Regression Model was used to identify factors that can predict the post-CI outcomes.
There was no significant difference in average scores between the two groups across five outcome measures, ranging from 88.40% to 95.65%. The correlation matrix revealed that younger ages at hearing aid fitting and CI activation positively influenced improvements in speech perception test scores. Furthermore, among the variables incorporated in the regression model, the duration of follow-up with CIs, age at CI activation, and the utilization of two CIs demonstrated prognostic significance for improved post-CI speech perception outcomes.
Conclusions
Children with ANSD can achieve similar open-set speech perception outcomes as children with SNHL. A longer CI follow-up, a lower age at CI activation, and the use of two CIs are predictive for optimal CI outcome.
Citation: Jafari Z, Fitzpatrick EM, Schramm DR, Rouillon I, Koravand A (2024) Predictors of cochlear implant outcomes in pediatric auditory neuropathy: A matched case-control study. PLoS ONE 19(5): e0304316. https://doi.org/10.1371/journal.pone.0304316
Editor: Renato S. Melo, UFPE: Universidade Federal de Pernambuco, BRAZIL
Received: February 26, 2024; Accepted: May 10, 2024; Published: May 29, 2024
Copyright: © 2024 Jafari et al. This is an open access article distributed under the terms of the Creative Commons Attribution License , which permits unrestricted use, distribution, and reproduction in any medium, provided the original author and source are credited.
Data Availability: The dataset used for this research belongs to the Children’s Hospital of Eastern Ontario (CHEO), Ottawa, ON, Canada. For this study, we did not have the ethical approval to upload a de-identified data set publicly. Data requests can be submitted to CHEO’s Research Ethics Board ( [email protected] ).
Funding: The author(s) received no specific funding for this work.
Competing interests: The authors have declared that no competing interests exist.
Introduction
Auditory neuropathy spectrum disorder (ANSD) is observed in 4% to 5% of all degrees of hearing loss, and 10% to 15% of school-age children with severe to profound sensorineural hearing loss (SNHL) [ 1 , 2 ]. ANSD is characterized by hearing impairment despite normally functioning outer hair cells (OHCs) [ 3 ]. In clinical audiology, ANSD is diagnosed by present otoacoustic emissions (OAE) and/or cochlear microphonics (CM), concomitant with the abnormal transmission of auditory signals from cochlear synapses to the brain as evidenced by absent or severely abnormal auditory brainstem responses (ABRs) [ 3 , 4 ]. The lesion site causing ANSD may vary from the presynaptic site of release of glutamate in the inner hair cells (IHCs) to the cochlear synapse, the postsynaptic site of neurotransmitter stimulation, the site of initiation of the excitatory postsynaptic potential (EPSP) at the terminal dendrite, or sites along the spiral ganglion, which affect auditory signal transmission along the auditory nerve to the brain [ 3 , 4 ]. In ANSD, deficiency in neural transmission (due to a reduced number of activated auditory nerve fibers or deafferentation) and/or neural dyssynchrony (due to progressive demyelination) are the two basic mechanisms that contribute to the disruption of neural activity and temporal resolution deficits at the auditory brainstem level [ 5 – 7 ]. Individuals with ANSD typically show difficulty with the temporal processing of sound resulting in impaired speech perception, especially in background noise [ 8 – 10 ].
The etiology of ANSD is complex and can broadly be classified into acquired and genetic factors [ 11 ]. A wide range of acquired factors, such as perinatal conditions, neurometabolic diseases, immune disorders, and ototoxic drug exposure might contribute to ANSD [ 11 – 13 ]. More than 40% of patients with auditory neuropathy have a genetic predisposition [ 11 ], including autosomal recessive (e.g., OTOF, PJVK), autosomal dominant (e.g., OPA1, MPZ, ATP1A3, SLC17A8, DIAPH3), X-linked (e.g., AIFM1), and mitochondrial mutations during maternal inheritance [ 14 , 15 ].
Intervention and management for ANSD are challenging and require a team approach including parental education about ANSD management [ 13 , 16 ]. Hearing technology interventions for ANSD vary according to individual cases due to the extent of the lesion and disease severity [ 17 ]. The American Academy of Audiology (AAA) Pediatric Amplification Guidelines recommend a hearing aid (HA) trial for children with reliable, permanent HL that interferes with speech perception at conversational levels [ 18 ]. However, HAs may not be an appropriate option for many patients with ANSD as making sounds louder does not improve the processing of auditory temporal cues [ 19 , 20 ]. Cochlear implantation has revolutionized the care for individuals with severe to profound hearing loss (HL). Given that the perception of auditory information is largely based on temporal processing, which can be successfully transmitted by only a few electrodes [ 21 ], cochlear implants (CIs) are the standard of care for many patients with ANSD [ 3 ]. The CI bypasses the lesion site (i.e., IHCs and cochlear synapses) and directly stimulates the spiral ganglion neurons. Further, compared to acoustic stimulation, electric stimulation is superior in stimulating and synchronizing auditory nerve fibers [ 3 ], which enhances neural synchrony and allows the development of fundamental speech and hearing skills [ 22 ].
Similar to children with SNHL [ 23 ], better outcomes in children with ANSD are associated with receiving hearing interventions (CIs or HAs) before three years of age [ 20 , 24 , 25 ]. While existing evidence demonstrates the benefits of CIs for both children with SNHL and ANSD [ 7 , 17 , 26 – 29 ], factors with prognostic value for intervention outcomes have been less studied [ 24 , 30 , 31 ]. This matched case-control study had two main objectives: 1) To compare children with ANSD and SNHL in open-set speech perception test scores through a matched case-control study design (1:2 ratio), and 2) To identify factors that show predictive value for post-CI speech perception outcomes. We hypothesized that with a matched case-control design, children with ANSD might achieve CI outcomes similar to peers with SNHL and that longer CI use and a lower age at CI activation might show prognostic value for optimal CI outcomes.
Participants
This retrospective case-control study was conducted at the Children’s Hospital of Eastern Ontario (CHEO) in Ottawa, Canada. Chart review and data extraction at CHEO was approved by the CHEO REB (Protocol No: 22/83X) with waived informed consent. All the data collected for this chart review were fully anonymized by the CHEO Research Group before granting access to the data. Additionally, the research received approval from the University of Ottawa Office of Research Ethics and Integrity (Ethics File Number # H-11-22-8149). The records of all children diagnosed with ANSD (n = 22), with CI outcome measures reported, between 01/09/2000 and 31/08/2022 were included in this study. Two research assistants, who were independent of the authors, conducted the data extraction. They were not privy to any information that could identify individual participants either during or after the data collection process. All children with ANSD included in this study exhibited bilateral auditory neuropathy, with no reported evidence of cochlear nerve deficiency (CND), as observed in MRI scans. For each child with ANSD, two children with SNHL with no evidence of cochleovestibular abnormalities were matched on biological sex, age of birth (months), age at CI activation, and length of using CIs. The ANSD diagnosis was based on inconsistency in audiologic findings in the clinical setting, consisting of present OAEs and/or CMs, absent or severely abnormal ABR, and various degrees of SNHL irrespective of present OAEs and/or CMs [ 32 ]. Children with less than six months of use of CIs were not included in the study. For data extraction, both electronic and paper records were reviewed by two research assistants independent of the authors. The list of data extracted for this study was: age of birth, HL characteristics (i.e., degree of HL and age of HL diagnosis), age at HA fitting, CI characteristics (i.e., type of CIs, the use of one or two CIs, and age at CI activation), medical conditions (additional disabilities [ADs], neonatal intensive care unit [NICU] history, and preterm birth [PB]), results of open-set speech perception test scores (i.e., outcome measures), and the length of follow-up with CIs.
Outcome measures
The outcome measures in this study consisted of tests of open-set speech recognition, including the Phonetically Balanced Kindergarten (PBK) test utilizing both monosyllabic word (PBK-w) and phoneme (PBK-p) speech materials, as well as the Hearing in Noise Test (HINT) for children employing sentence materials in both quiet and noise conditions. For the PBK test, recorded speech materials comprising 25 monosyllabic words (PBK-w) and 80 phonemes (PBK-p) were presented at 60 dB SPL [ 33 ]. The HINT test sentences were administered in both quiet and noise conditions with speech-shaped noise at 10- and 5-dB signal-to-noise ratios (SNRs: HINT-10dB and HINT-5dB, respectively) at 60 dB SPL [ 34 ]. All speech perception tests were conducted within a sound booth in the clinical setting. In the chart review, the length of follow-up with CIs was defined based on the most recent follow-up session with outcome measures reported. Therefore, this measure referred to the difference between the date of CI activation and the date of speech test administration.
Statistical analysis
Statistical analysis was conducted using SPSS Statistics 26.0 at a significance level of 0.05 or better. The univariate analysis of variance test was utilized to compare the two groups in speech perception outcomes. The Spearman’s rank correlation coefficient test was employed to construct the Correlation Matrix. A forward linear multiple regression model was performed to ascertain the variables that had predictive value for post-CI speech perception outcomes.
The present retrospective study reports the results of 66 children with CIs including 22 with ANSD (13 [59.10%] males and 9 [40.90%] females) and 44 children with SNHL (26 [59.10%] males and 18 [40.90%] females) aged 5 to 18 years old. Table 1 demonstrates demographic information for the two study groups including average age of birth (months), age at HL diagnosis, age at HA fitting, age at CI activation, biological sex, the use of one or two CIs, ADs/MCs (such as developmental delay, attention deficit hyperactivity disorder, autism, asthma, dyslexia, visual impairment, cerebral palsy, and cytomegalovirus), and the severity of HL. The two groups were not significantly different in age at HL diagnosis (p = 0.645), age at HA fitting (p = 0.953), age at CI activation (p = 0.484), and length of follow-up period (p = 0.389) ( Table 1 ).
- PPT PowerPoint slide
- PNG larger image
- TIFF original image
https://doi.org/10.1371/journal.pone.0304316.t001
Open-set speech perception test results
No significant difference was observed between the SNHL and ANSD groups in the results of outcome measures ( Fig 1 ), consisting of PBK-w test (F = 0.126, p = 0.724, partial η 2 = 0.002, power = 0.064), PBK-p test (F = 0.054, p = 0.817, partial η 2 = 0.001, power = 0.056), HINT test (F = 0.018, p = 0.892, partial η 2 = 0.001, power = 0.052), HINT-10dB test (F = 0.298, p = 0.588, partial η 2 = 0.012, power = 0.131), and HINT-5dB test (F = 0.054, p = 0.817, partial η 2 = 0.006, power = 0.083).
No significant difference was found between the two groups. The bars exhibit mean ± 2SEM (standard error of the mean). ANSD, auditory neuropathy spectrum disorders; SNHL, sensorineural hearing loss.
https://doi.org/10.1371/journal.pone.0304316.g001
Correlation matrix
Fig 2 displays the Correlation Matrix, illustrating the strength of Spearman correlations between three critical ages (age of HL diagnosis, HA fitting, and CI activation) and the results of five open-set speech perception test scores (outcome measures). Each block represents the correlation between two variables and the associated p-value. Darker shades of blue and red indicate stronger positive and negative correlations, respectively.
Each block shows the correlation between two variables and the associated p-value. Darker blue and red colors refer to greater positive and negative associations, respectively. HINT, Hearing in Noise Test (HINT-5dB and HINT-10dB); PBK-w/p, Phonetically Balanced Kindergarten with words or phonemes.
https://doi.org/10.1371/journal.pone.0304316.g002
Predictors of intervention outcomes
A Forward Linear Multiple Regression Model was applied to determine factors that might have predictive value for the post-CI outcomes. The variables included in the model were age at HL diagnosis, age at HA fitting, age at CI activation, the length of follow-up with CIs, the use of one or two CIs, history of NICU admission and PB, presence of ADs, and biological sex. Table 2 summarizes the results of statistical analyses, including the regression coefficient (R square), F and p values, and standardized coefficient Beta. For example, among the variables included in the regression model, “the length of follow-up with CIs” was the only factor that showed a significant contribution (r = 0.328, p ≤ 0.001) to the PBK-w test results. Based on the regression coefficient, it could be interpreted that 32.80% of the outcome (i.e., the PBK-W test score) was explained by the length of follow-up with CIs. In addition, the positive magnitude of the beta value (0.531) indicated improved speech perception performance with increased length of CI use. The same results were obtained for the PBK-p test, corroborating the predictive value of the length of CI use for post-CI speech perception outcomes. For the HINT in quiet and HINT-10dB tests, the model identified two predictors of the outcomes. In addition to the length of follow-up with CIs, adding the “age at CI activation” significantly improved the predictive value of the model. However, for the HINT-5dB test, “bilateral amplification” was the factor that showed significant prognostic value.
https://doi.org/10.1371/journal.pone.0304316.t002
The present study had three primary findings: 1) The ANSD and SNHL groups achieved high scores in post-CI speech perception outcomes, with no significant difference observed between the two groups. 2) Speech perception test scores were positively associated with a lower age at HA fitting and at CI activation and a longer follow-up with CIs. 3) Three variables including a longer use of CIs, age at CI activation, and the use of two CIs showed a predictive value for better speech perception outcomes. In the following paragraphs, the main findings are discussed.
Post-CI open-set speech perception outcomes
In our study, both the ANSD and SNHL groups achieved optimal CI outcomes across five open-set speech perception test scores, in both quiet (PBK-w, PBK-p, and HINT in quiet) and noise (HINT-5dB and HINT-10dB) conditions, with no notable difference between the two groups. Children with ANSD displayed no indications of CND in MRI scan reports, suggesting a potential involvement of presynaptic mechanisms in ANSD. This finding is consistent with past studies indicating that the majority of children with presynaptic ANSD can attain speech understanding, language development, and communication outcomes equivalent to their peers with SNHL [ 7 , 28 ]. However, children with postsynaptic ANSD, comprising almost 30% of children with ANSD [ 13 ], exhibit limited benefits from CIs and cannot develop functionally useful auditory communication skills [ 26 , 27 ]. Thus, in ANSD, the lesion site along the auditory pathway carries prognostic significance, in which individuals with presynaptic ANSD or distal auditory nerve lesions (such as the involvement of inner hair cells or cochlear synapses) achieve optimal outcomes compared to children with postsynaptic involvement [ 3 , 7 , 22 ].
Correlation matrix findings
As presented in the correlation matrix, a mild to high association was found between the results of speech perception test scores, with a lower magnitude of correlations between HINT-5dB with speech tests conducted in quiet. This finding is aligned with current evidence indicating speech perception in noise, especially at lower SNRs, as the most challenging listening condition for individuals with hearing loss [ 35 ], including children with cochlear implants [ 36 – 38 ]. Hearing-impaired individuals are highly noise intolerant, and despite remarkable improvements in current HA and CI technology, existing devices may not be very effective at combating background noise [ 39 ].
In the present study, open-set speech perception test scores were negatively associated with longer ages at HL diagnosis, HA fitting, and CI activation and positively associated with the length of follow-up with CIs (i.e., longer use of CIs). Our findings are aligned with past evidence regarding the impact of auditory deprivation and longer durations of HA or CI usage on spoken language development. For example, in two studies [ 40 , 41 ], children with ANSD who received CIs before 24 months of age achieved higher scores in the Categories of Auditory Perception (CAP) test and Speech Intelligibility Rating (SIR) test compared to children who were implanted after 24 months of age. In a systematic review with a narrative synthesis of evidence [ 42 ] on implanted children with follow-up periods ranging from 6 months to 9 years, cochlear implantation before 24 months was found to be beneficial based on the scores of PBK and consonant-nucleus-consonant (CNC) tests. In addition, implantation before 12 months was associated with better speech production (using Diagnostic Evaluation of Articulation and Phonology and Infant-Toddler Meaningful Auditory Integration Scale [IT-MAIS]), auditory performance (the CAP-II score), and receptive language scores (based on the Preschool Language Scale combined with oral and written language skills and Peabody Picture Vocabulary Test). In another similar review, language outcomes for children implanted after 12 months decreased with the increased age of implantation [ 43 ]. In a study investigating cortical maturation, measured by P1 cortical auditory evoked potential (CAEP) latency, P1 CAEP latency was significantly correlated with children’s scores on the IT-MAIS. P1 CAEP responses were present in all children after implantation [ 44 ], compared to previous studies suggesting that only 50 [ 45 ] to 75 [ 46 ] of ANSD children with HAs showed CAEP responses. It was concluded that children fitted with CIs under two years of age were more likely to show age appropriate CAEP responses within six months after implantation, suggesting a possible sensitive period for cortical auditory development in ANSD [ 44 ].
The importance of early HL diagnosis and early hearing intervention is associated with the fact that the brain has high synaptic plasticity during childhood that progressively declines with age. This decline results from developmental mechanisms such as attenuated synaptic conductivity and the maturation of inhibitory neurons [ 47 ]. This age-related neural development shapes sensory object discrimination [ 48 ]. In addition, brain development is highly modulated by sensory inputs and profoundly reshaped by the lack of one sensory modality [ 49 , 50 ]. According to MRI studies, early auditory deprivation leads to reduced white matter volume and integrity in the primary and secondary auditory cortex and spoken-language brain areas [ 51 , 52 ]. The extent of structural neuroplasticity is an index of poor speech-language performance in late CI recipients [ 53 ]. In addition, auditory processing deficits due to functional intra-modal changes (e.g., the reduction of tonotopy, dynamic range, temporal resolution, and sensitivity to binaural cues) occur, which severely degrade the acuity of the auditory signal perceived [ 48 , 54 ]. The findings of studies reviewed here underscore the crucial role of the sensitive period of auditory and spoken language development; a time limit of below 4 years, especially within the first two years of life [ 55 – 57 ], in which the central auditory system is highly plastic, and CI surgery could result in optimal outcomes [ 48 ].
Factors with prognostic value for speech perception outcomes
We used a forward multiple regression model to determine the factors showing significant prognostic value for post-intervention outcomes. Among the variables included in the regression model, a longer follow-up with CIs, a lower age at CI activation, and the use of two CIs were the three factors that showed predictive value for optimal speech perception outcomes. Limited studies have reported the use of statistical models in identifying predictors of post-CI outcomes. In a prospective study by Ching et al. (2013) on 451 children in Australia with HL (30% with CIs, 10% with ANSD), age at CI activation, absence of ADs, higher maternal education, and female sex were reported as predictors for post-CI outcomes [ 24 ]. In a subsequent report by the same research group on language outcomes [ 58 ], the benefit of early intervention for language development increased as hearing loss increased. Children who received amplification at age 24 months had lower language scores than those fitted at 3 months, and children who received CIs at 24 months had lower language scores than those implanted at 6 months. In a retrospective study by le Roux et al. (2016) on 301 children with CIs (3.5% with ANSD) from five CI programs in South Africa, using two CIs, ADs/MCs, mainstream education, and ethnicities other than Caucasian contributed to post-CI outcomes [ 30 ].
An interesting finding in the present study was the significant contribution of the length of follow-up with CIs to the PBK (word and phoneme) and HINT in quiet and SNR 10dB test scores and the use of two CIs to HINT-5dB test scores. These findings underscore the crucial role of binaural hearing in speech perception, particularly in noisy environments. Even individuals with normal hearing find understanding speech in noise challenging [ 59 ]. Binaural hearing can substantially improve speech perception in noisy conditions and has been the focus of several trials for users of hearing technologies [ 60 – 62 ]. The binaural neural processing of the interaural level difference (ILD) and interaural time difference (ITD) can improve the internal SNR and consequently speech intelligibility [ 59 , 63 ].
This study had two major strengths compared to past publications in the field. Firstly, employing a matched case-control study design on several factors contributing to CI outcomes allowed us to conduct a more precise comparison between children with ANSD and peers with SNHL. By matching the two groups, we not only observed similar progress and achievement of optimal CI outcomes in children with ANSD compared to the control group but also noted that ANSD was not identified as a predictor of the outcomes in the Forward Regression Model. This indicates similar speech perception progress with CIs in ANSD and SNHL under matched conditions. Secondly, this study was one of a few research efforts with a proper design reporting the factors with predictive value for optimal CI outcomes. The study’s limitation was a lack of information about other potential contributors, such as electrophysiological findings [ 31 ], maternal education, mode of communication at home, and socioeconomic status, which could affect the outcomes and their potential impact should be taken into account [ 24 , 30 , 58 , 64 ]. Furthermore, the dataset used for this retrospective study did not encompass electrophysiological (e.g., electric auditory evoked potentials [eABR]) and genetic findings, which could offer insights into potential etiologies and enhance conclusions.
In this retrospective matched (1:2) case-control study, we investigated post-CI speech perception outcomes in children with ANSD compared to peers with SNHL. Our findings revealed that under matched conditions, children with ANSD (without indications of postsynaptic involvement in MRI) achieved CI outcomes comparable to children with SNHL. This study contributes to the limited body of literature identifying factors predictive of post-CI outcomes. Utilizing a regression model, we identified three significant predictors of speech perception outcomes: duration of CI use, age at CI activation, and the utilization of bilateral CIs. Future research should consider additional potential contributors, such as genetics, socioeconomic status, and cognitive factors, to develop a more comprehensive model elucidating the determinants of post-CI speech perception outcomes.
Supporting information
https://doi.org/10.1371/journal.pone.0304316.s001
https://doi.org/10.1371/journal.pone.0304316.s002
Acknowledgments
This article was part of a Ph.D. dissertation project in Rehabilitation Sciences, the Audiology and Speech-Language Pathology Program, approved by Ottawa University, Faculty of Health Sciences, School of Rehabilitation Sciences. We appreciate Chantal Lessard (Coordinator and Professional Practice Leader at CHEO) and JoAnne Whittingham (Research Coordinator at CHEO) who helped with ethical approval and access to data and Ines Telmat and Jennifer Ti Nguyen who assisted with data extraction.
- View Article
- PubMed/NCBI
- Google Scholar
- 5. Rance G, Starr A. Auditory neuropathy/dys-synchrony. In: Seewald R, Tharpe A, editors. Comprehensive handbook of pediatric audiology San Diego: Plural Publishing; 2011. p. 225–42.
- 6. Starr A, Rance G. Auditory neuropathy. In: Celesia G, Hickok G, editors. Handbook of Clinical Neurology. 129. Edinburgh Elsevier; 2015. p. 495–508.
- 13. Hall J. eHandbook of Auditory Evoked Responses: Principles, Procedures & Protocols: Pearson Education, Inc.; 2015.
- 16. Wolfe J. Cochlear Implants: Audiologic Management and Considerations for Implantable Hearing Devices. San Diego: Plural Publishing Inc.; 2020.
- 32. Hayes D, Sininger Y. Guidelines for Identification and Management of Infants and Young Children with Auditory Neuropathy Spectrum Disorder. NHS 2008, Como, Italy2008.
Thank you for visiting nature.com. You are using a browser version with limited support for CSS. To obtain the best experience, we recommend you use a more up to date browser (or turn off compatibility mode in Internet Explorer). In the meantime, to ensure continued support, we are displaying the site without styles and JavaScript.
- View all journals
- My Account Login
- Explore content
- About the journal
- Publish with us
- Sign up for alerts
- Review Article
- Open access
- Published: 08 April 2024
The evolution of robotics: research and application progress of dental implant robotic systems
- Chen Liu ORCID: orcid.org/0009-0000-1771-5430 1 , 2 , 3 , 4 na1 ,
- Yuchen Liu 1 , 2 , 3 , 4 na1 ,
- Rui Xie 1 , 2 , 3 , 4 ,
- Zhiwen Li 1 , 2 , 3 , 4 ,
- Shizhu Bai ORCID: orcid.org/0000-0002-2439-3211 1 , 2 , 3 , 4 &
- Yimin Zhao 1 , 2 , 3 , 4
International Journal of Oral Science volume 16 , Article number: 28 ( 2024 ) Cite this article
1550 Accesses
17 Altmetric
Metrics details
- Medical research
- Oral diseases
- Preclinical research
The use of robots to augment human capabilities and assist in work has long been an aspiration. Robotics has been developing since the 1960s when the first industrial robot was introduced. As technology has advanced, robotic-assisted surgery has shown numerous advantages, including more precision, efficiency, minimal invasiveness, and safety than is possible with conventional techniques, which are research hotspots and cutting-edge trends. This article reviewed the history of medical robot development and seminal research papers about current research progress. Taking the autonomous dental implant robotic system as an example, the advantages and prospects of medical robotic systems would be discussed which would provide a reference for future research.
Similar content being viewed by others
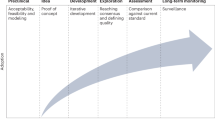
The IDEAL framework for surgical robotics: development, comparative evaluation and long-term monitoring
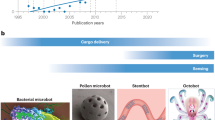
Clinical translation of wireless soft robotic medical devices
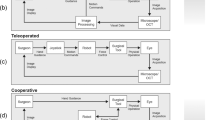
Advanced robotic surgical systems in ophthalmology
The development of medical robots has been a long journey of exploration. After being practically validated in industrial robots, this technology has become widespread globally and is now an essential part of modern production and lifestyles. Medical robots are increasingly in the vanguard of the field in diagnosis, treatment, visualization, and other areas of clinical practice. We are currently witnessing a transformative shift from cutting-edge research to the widespread application of medical robots. This review focused on the historical trajectory of medical robots, with a particular emphasis on the development history, current research status, and prospects of dental implant robotic systems.
Definition and history of robots
Definition and architectures of robots.
According to the International Organization for Standardization (ISO), a robot is an automatic, position-controlled, programmable multi-functional manipulator with several axes. It can process various materials, parts, tools, and special devices through programmable automation to perform intended tasks. 1 A robot’s structure typically consists of four parts: the actuation system, the drive-transmission system, the control system, and the intelligent system. The actuation system is the part of the robot that directly performs work, similar to a human hand. The drive-transmission system transmits force and motion to the actuator through a power source. The control system comprises a control computer, control software, and servo controllers, similar to a human brain. The intelligent system typically includes a perception system and an analytical decision-making intelligent system.
Evolution of robots
The history of robots can be traced back over 3 000 years. 2 Throughout history, scientists and craftsmen have designed and manufactured robot prototypes that simulate animal or human characteristics. 1 However, these inventions can only be classified as mechanical devices that primarily achieved automated functions through mechanical and physical principles with the lack of intelligence and autonomy of modern robots. These inventions demonstrate the level of engineering technology and mechanical manufacturing in ancient times, laying the foundation for later research on robots. Joseph Engelberger, recognized as the Father of Robotics, founded Unimation Corporation in 1958, the world’s first robot-manufacturing factory, which marked the official start of the industrialization of robots. In 1978, Unimation developed a Programmable Universal Machine for Assembly (PUMA) which represents a significant milestone in the development of international industrial robotics. In recent years, robotics has expanded significantly due to the continued development of sensor types, intelligent algorithms, and multidisciplinary integration. The technology has advanced from the initial industrial robotic arms to bionic robots, soft robots, nanorobots, and other forms.
Classification of robotics
The International Federation of Robotics (IFR) classifies robotics into two distinct categories: industrial robotics and service robotics, in accordance with the international standard ISO 8373:2012. 3 Industrial robotics are multipurpose manipulators with automatic control and programmability, which can operate with fixed or autonomous mobility and are primarily used in industrial production. 3 Service robotics are driving mechanisms that can perform useful tasks but do not include industrial automation applications. The IFR has classified service robotics into different segments to meet the diverse requirements of various industries (Fig. 1 ).
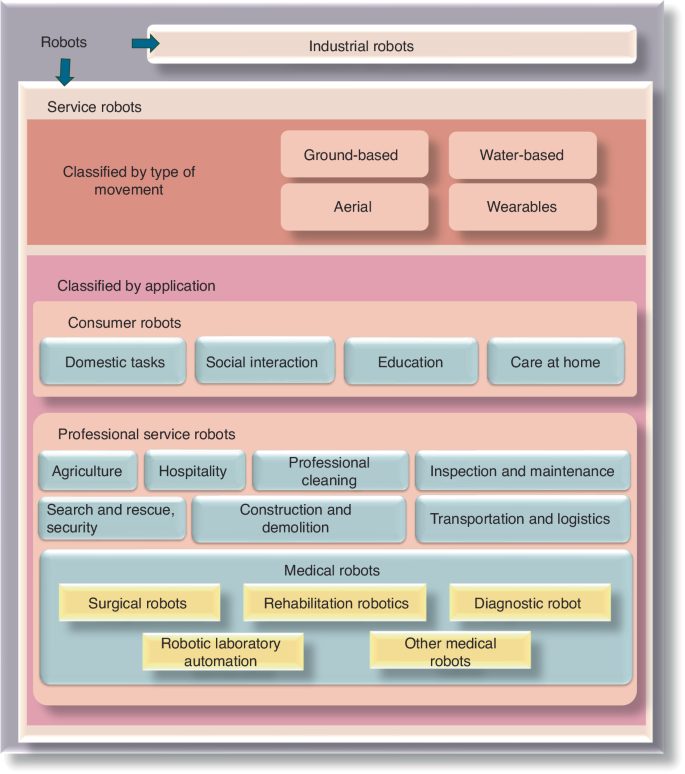
Categories of robots according to the International Federation of Robotics
Medical robotics
In 1985, the Puma 200 robot (Westinghouse Electric, Pittsburgh, PA) was used for needle placement in computed tomography (CT)-guided brain biopsy at the Los Angeles Hospital in the United States, marking the beginning of the era of medical robot applications. 4 , 5 After nearly 40 years of continuous development and progress, medical robotics have been widely used in multiple fields, including surgery, nursing, and rehabilitation, demonstrating numerous remarkable advantages and potential.
Yang 6 , 7 , 8 has divided the level of autonomy of medical robotics into six levels, as follows: (0) no autonomy, (1) robot assistance, (2) task autonomy, (3) conditional autonomy, (4) high autonomy, and (5) full autonomy. At level 0, the robot requires operators to perform all tasks, including monitoring, generating performance options, selecting the option to perform (decision making), and executing the decision made, such as the da Vinci robotic system (Intuitive Inc., California, USA). At level 1, operators are required to continuously control the robot while the robot provides guidance with positional constraints. The Mako Smart Robotics used in orthopedic surgery is an example. At level 2, operators are required to discretely rather than continuously control the robot, and the robot can independently complete specific tasks based on operator instructions and pre-programmed procedures. An example of this level is the ROBODOC, which performs total hip and total knee replacement surgeries. At level 3, robots have the ability to perform surgeries based on pre-programmed procedures and can also modify the pre-planned schedule in real time to accommodate changes in the intraoperative position of the target object. An example of such robotics is the CyberKnife radiation therapy robotics, which has respiratory tracking functionality. At the higher levels of autonomy (specifically level 5 and possibly level 4), the robot is not only a medical device but also capable of practicing medicine, which currently does not exist due to some regulatory, ethical, and legal considerations. 6 , 7 , 8
Medical robotics are classified by IFR as special robotics with a combination of medical diagnosis methods with new technologies, such as artificial intelligence (AI) and big data, to provide services such as surgery, rehabilitation, nursing, medical transportation, and consultation for patients. 9 Medical robotics are categorized into the following five types based on their functions: surgical robotics, rehabilitation robotics, diagnostic robotics, laboratory analysis automation, and other robotics (robotics used for medical transportation are not included in this category).
Surgical robotics
Minimally invasive surgery and accurate intervention require surgeons to exercise more discernment, expand their range of vision, and increase their flexibility which brings the surgical robotics development (the surgical robot architecture 10 was shown in Fig. 2 ). Not only can it be equipped with an advanced three-dimensional (3D) imaging system and augmented reality technology to provide high-definition images of the surgical scene, but it is also capable of displaying important anatomical structures such as blood vessel and nerve locations in real-time. This allows surgeons to perform precise operations with the assistance of robots. For higher-level automatic medical robots, precise surgical operations are performed through image guidance and navigation systems based on preoperative planning. Moreover, the robotic arm has a high level of precision and stability that surpasses the capabilities of a free hand. This allows it to perform small and delicate operations with reduced errors caused by physician experience, fatigue, and hand tremors. In addition, the surgical robot also integrates artificial intelligence technology, which can perform automatic diagnostic analysis, adjust surgical strategies, and provide personalized surgical plans through deep learning. 11 Therefore, surgical robots could utilize vision, speech recognition, telecommunication, 3D imaging, and artificial intelligence technologies to enhance surgical skills through sensing and image guidance systems. This overcomes the limitations of manual operations and improves surgical accuracy and reliability. In comparison to conventional surgery, robotic-assisted surgery could reduce trauma, shorten recovery periods, and relieve pain. 12 , 13 Additionally, it can be used for remote surgery, operates continuously without fatigue, reduces the workload of medical staff, and minimizes occupational exposure for surgeons. Medical robotics have gradually entered the commercialization stage and have been utilized in clinical settings (Table 1 ). Currently, the most well-known surgical robot is the da Vinci system, which enables surgeons to accurately and minimally perform invasive surgery for multiple complicated diseases with good hand-eye coordination and magnification.
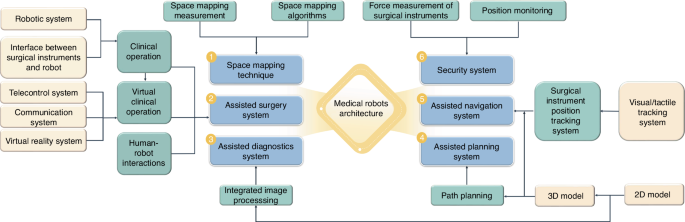
The surgical robotic architecture
Dental treatment involves the special anatomical structure of the mouth and is characterized by limited visibility, narrow operation space, and the disturbance of saliva and tongue. As a result, the dental operation is intricate and mainly reliant on the surgeon’s experience and expertize, which takes inexperienced surgeons a long time to acquire. With the successful use of the da Vinci robotic system in laparoscopic surgery, surgeons are beginning to consider its potential application in maxillofacial surgery. Da Vinci robot has been used for cleft palate repair, 14 , 15 treating patients with obstructive sleep apnea-hypopnea syndrome (OSAHS), 16 as well as oral and oropharyngeal tumor resection. 17 , 18 However, due to the complexity of the oropharyngeal anatomy, the multiple robotic arms of the da Vinci system limit the surgeon’s vision, which is not conducive to surgical performance. In order to overcome these shortcomings, flexible robots (such as The Flex) approved by the Food and Drug Administration have made it possible to be used for oropharyngeal surgery. Additionally, oral and cranio-maxillofacial bone surgery, such as orthognathic surgery and dental implant surgery, requires accurate ostomies, which cannot be achieved by the da Vinci system. Robotic-assisted dental implant surgery research originated in 2001, and related studies have shown a gradual increase in recent years. In addition to conventional implant surgery, dental implant robotics can also perform zygomatic implant placement. 19 , 20 Among these studies, the largest number of articles were published in China, followed by the United States (Fig. 3 ). In Part 3 of this article, the relevant studies on dental implant robotics will be elaborated in detail.
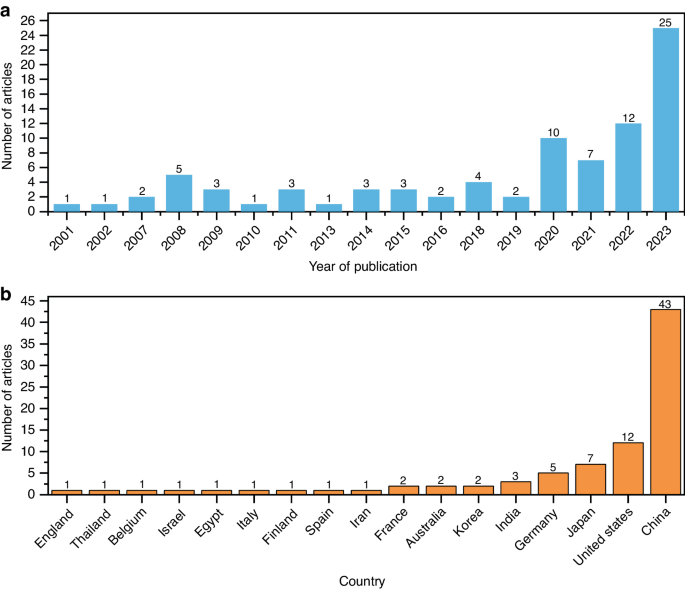
Related research on robotic-assisted dental implant placement. a The number of published papers on dental implant robotics in different years and b in different countries (as of December 2023)
Rehabilitation robotics
Rehabilitation robotics are significant area and research hotspot in medical robotics, second only to surgical robotics. Rehabilitation robotics are classified into two categories, as follows: therapeutic and assistive robotics. Therapeutic robotics provide psychological or physical treatment to improve specific functions of patients and are widely used in physical training and functional recovery of patients with paralysis and in improving the interactive ability of children with autism through behavioral induction. 21 Assistive robotics aim to improve the quality of life for individuals with musculoskeletal or neuromuscular impairments by compensating for or replacing their mobility or functionality. 22 , 23 , 24 For instance, Mike Topping’s Handy1 assists the most severely disabled with several everyday functions. 25 Similarly, Israel’s ReWalk provides powered hip and knee motion to enable individuals with spinal cord injury to stand upright, walk, turn, climb, and descend stairs. 26 Moreover, Japan’s wearable powered prosthesis, HAL, can enable patients to control joint movements independently by detecting bioelectrical signals on the skin surface during movement, in combination with foot pressure sensors. 27
Diagnostic robotics
Diagnostic robotics aid doctors in conducting examinations and making diagnoses, with the aim of improving accuracy, convenience, non-invasiveness, and safety of diagnosis. For instance, wireless capsule endoscopy introduced by Given Imaging (now Medtronic) allows minimally invasive inspection of the gastrointestinal tract. Patients can swallow a pillcam that captures images deep within the intestines, which has revolutionized gastrointestinal endoscopy and is now a clinically viable alternative to standard interventional endoscopy. Furthermore, wearable robotics are increasingly being utilized to non-invasively detect various health indicators and assist in disease diagnosis.
Laboratory robotics
Laboratory robotics handle and analyze samples in medical laboratories. Innovations in robotics and information technologies have created new opportunities for laboratory automation. These robots tirelessly and accurately perform tasks, improving the precision and reliability of experiments while reducing costs. At the University of Virginia Medical Center, robots operate instruments and analyze blood gases and electrolytes in the hospital laboratory. In addition, the robotic system works continuously, not only improving laboratory efficiency but also reducing the burden on laboratory techniques. 28 Nicole Rupp, based in Germany, has utilized the Dobot Magician robot to develop an economical automated laboratory system that coordinates various instruments for experiments. The results obtained from this system were not statistically different from those obtained from manual experiments. 29
Other medical robotics
The medical field has witnessed a significant increase in the use of robotics, leading to the development of new types of robots and functions to cater to the requirements of doctors and patients. Other medical robotics include providing non-medical operational services, such as assisting nurses with guidance, transportation, cleaning, inspection, monitoring, and disinfection. Moreover, robotics could be available for daily home care, providing assistance, monitoring behavior and health, as well as offering companionship for older individuals. 30 Furthermore, there are robots specifically designed to train emergency personnel. These robots can simulate complex trauma scenarios with multiple injuries in a highly accurate manner. 31 Robotic surgery simulation practice can be combined with virtual reality (VR), 3D-printed organ tissue models, or anesthetized live animals to rapidly improve the robotic surgical skills required by novice surgeons. In addition, to pandemics such as Ebola and COVID-19, the use of sampling robotics can effectively reduce the risk of infection. There are robots also designed for emergency rescue, medical education, and training. 32 , 33 Soft robotics, bionic robotics, nanorobots, and other robotics suitable for various functional needs are also hot topics in current medical robotic research, and they exhibit the typical characteristics of specialization, personalization, remoteness, intelligence, and immersion.
Dental implant robotic system
Implantology is widely considered the preferred treatment for patients with partial or complete edentulous arches. 34 , 35 The success of the surgery in achieving good esthetic and functional outcomes is directly related to correct and prosthetically-driven implant placement. 36 Accurate implant placement is crucial to avoid potential complications such as excessive lateral forces, prosthetic misalignment, food impaction, secondary bone resorption, and peri-implantitis. 37 Any deviation during the implant placement can result in damage to the surrounding blood vessels, nerves, and adjacent tooth roots and even cause sinus perforation. 38 Therefore, preoperative planning must be implemented intraoperatively with utmost precision to ensure quality and minimize intraoperative and postoperative side effects. 39
Currently, implant treatment approaches are as follows: Free-handed implant placement, Static computer-aided implant placement, and dynamic computer-aided implant placement. The widely used free-handed implant placement provides less predictable accuracy and depends on the surgeon’s experience and expertise. 40 Deviation in implant placement is relatively large among surgeons with different levels of experience. When novice surgeons face complex cases, achieving satisfactory results can be challenging. A systematic review 41 based on six clinical studies indicated that the ranges of deviation of the platform, apex, and angle from the planned position with free-handed implant placement were (1.25 ± 0.62) mm–(2.77 ± 1.54) mm, (2.10 ± 1.00) mm–(2.91 ± 1.52) mm, and 6.90°± 4.40°–9.92°± 6.01°, respectively. Static guides could only provide accurate guidance for the initial implantation position. However, it is difficult to precisely control the depth and angle of osteotomies. 42 The lack of real-time feedback on drill positioning during surgery can limit the clinician’s ability to obtain necessary information. 42 , 43 , 44 Besides, surgical guides may also inhibit the cooling of the drills used for implant bed preparation, which may result in necrosis of the overheated bone. Moreover, the use of static guides is limited in patients with limited accessibility, especially for those with implants placed in the posterior area. Additionally, the use of guides cannot flexibly adjust the implant plan intraoperatively. With dynamic computer-aided implant placement, the positions of the patient and drills could be tracked in real-time and displayed on a computer screen along with the surgical plan, thus allowing the surgeon to adjust the drilling path if necessary. However, the surgeons may deviate from the plan or prepare beyond it without physical constraints. During surgery, the surgeon may focus more on the screen for visual information rather than the surgical site, which can lead to reduced tactile feedback. 45 The results of a meta-analysis showed that the platform deviation, apex deviation, and angular deviation were 0.91 mm (95% CI 0.79–1.03 mm), 1.26 mm (95% CI 1.14–1.38 mm), and 3.25° (95% CI 2.84°–3.66°) respectively with the static computer-aided implant placement, and 1.28 mm (95% CI 0.87–1.69 mm), 1.68 mm (95% CI 1.45–1.90 mm), and 3.79° (95% CI 1.87–5.70°), respectively, with dynamic computer-aided implant placement. The analysis results showed that both methods improved the accuracy compared to free-handed implant placement, but they still did not achieve ideal accuracy. 46 Gwangho et al. 47 believe that the key point of a surgical operation is still manually completed by surgeons, regardless of static guide or dynamic navigation, and the human factors (such as hand tremble, fatigue, and unskilled operation techniques) also affect the accuracy of implant placement.
Robotic-assisted implant surgery could provide accurate implant placement and help the surgeon control handpieces to avoid dangerous tool excursions during surgery. 48 Furthermore, compared to manual calibration, registration, and surgery execution, automatic calibration, registration, and drilling using the dental implant robotic system reduces human error factors. This, in turn, helps avoid deviations caused by surgeons’ factors, thereby enhancing surgical accuracy, safety, success rates, and efficiency while also reducing patient trauma. 7 With the continuous improvement of technology and reduction of costs, implant robotics are gradually becoming available for commercial use. Yomi (Neocis Inc., USA) has been approved by the Food and Drug Administration, while Yakebot (Yakebot Technology Co., Ltd., Beijing, China), Remebot (Baihui Weikang Technology Co., Ltd, Beijing, China), Cobot (Langyue dental surgery robot, Shecheng Co. Ltd., Shanghai, China), Theta (Hangzhou Jianjia robot Co., Ltd., Hangzhou, China), and Dcarer (Dcarer Medical Technology Co., Ltd, Suzhou, China) have been approved by the NMPA. Dencore (Lancet Robotics Co., Ltd., Hangzhou, China) is in the clinical trial stage in China.
Basic research on dental implant robotic system
Compared to other surgeries performed with general anesthesia, dental implant surgery can be completed under local anesthesia, with patients awake but unable to remain completely still throughout the entire procedure. Therefore, research related to dental implant robotic system, as one of the cutting-edge technologies, mainly focuses on acquiring intraoperative feedback information (including tactile and visual information), different surgical methods (automatic drilling and manual drilling), patient position following, and the simulation of surgeons’ tactile sensation.
Architecture of dental implant robotic system
The architecture of dental implant robotics primarily comprises the hardware utilized for surgical data acquisition and surgical execution (Fig. 4 ). Data acquisition involves perceiving, identifying, and understanding the surroundings and the information required for task execution through the encoders, tactile sensors, force sensors, and vision systems. Real-time information obtained also includes the robot’s surrounding environment, object positions, shapes, sizes, surface features, and other relevant information. The perception system assists the robot in comprehending its working environment and facilitates corresponding decision-making as well as actions.
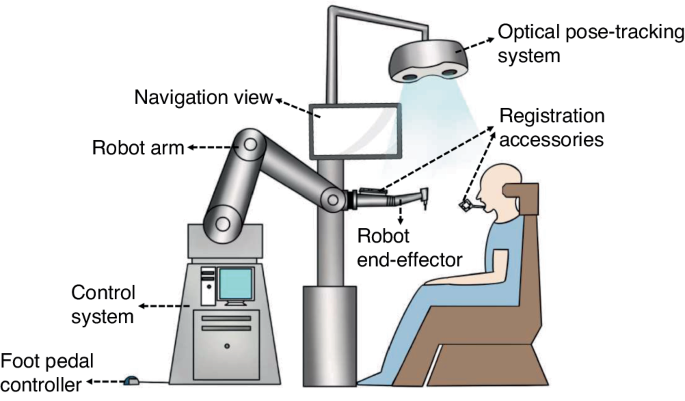
The architecture of dental implant robotics
During the initial stage of research on implant robotics, owing to the lack of sensory systems, fiducial markers and corresponding algorithms were used to calculate the transformation relationship between the robot’s and the model’s coordinate system. The robot was able to determine the actual position through coordinate conversions. Dutreuil et al. 49 proposed a new method for creating static guides on casts using robots based on the determined implant position. Subsequently, Boesecke et al. 50 developed a surgical planning method using linear interpolation between start and end points, as well as intermediate points. The surgeon performed the osteotomies by holding the handpieces, with the robot guidance based on preoperatively determined implant position. Sun et al. 51 and McKenzie et al. 52 registered cone-beam computed tomography (CBCT) images, the robot’s coordinate system, and the patient’s position using a coordinate measuring machine, which facilitated the transformation of preoperative implant planning into intraoperative actions.
Neocis has developed a dental implant robot system called Yomi (Neocis Inc.) 53 based on haptic perception and connects a mechanical joint measurement arm to the patient’s teeth to track their position. The joint encoder provides information on the drill position, while the haptic feedback of handpieces maneuvered by the surgeon constrains the direction and depth of implant placement.
Optical positioning is a commonly used localization method that offers high precision, a wide -field -of -view, and resistance to interference. 54 This makes it capable of providing accurate surgical guidance for robotics. Yu et al. 55 combined image-guided technology with robotic systems. They used a binocular camera to capture two images of the same target, extract pixel positions, and employ triangulation to obtain three-dimensional coordinates. This enabled perception of the relative positional relationship between the end-effector and the surrounding environment. Yeotikar et al. 56 suggested mounting a camera on the end-effector of the robotic arm, positioned as close to the drill as possible. By aligning the camera’s center with the drill’s line of sight at a specific height on the lower jaw surface, the camera’s center accurately aligns with the drill’s position in a two-dimensional space at a fixed height from the lower jaw. This alignment guides the robotic arm in drilling through specific anatomical landmarks in the oral cavity. Yan et al. 57 proposed that the use of “eye-in-hand” optical navigation systems during surgery may introduce errors when changing the handpiece at the end of the robotic arm. Additionally, owing to the narrow oral environment, customized markers may fall outside the camera’s field of view when the robotic arm moves to certain positions. 42 To tackle this problem, a dental implant robot system based on optical marker spatial registration and probe positioning strategies is designed. Zhao et al constructed a modular implant robotic system based on binocular visual navigation devices operating on the principles of visible light with “eye-to-hand” mode, allowing complete observation of markers and handpieces within the camera’s field of view, thereby ensuring greater flexibility and stability. 38 , 58
The dental implant robotics execution system comprises hardware such as motors, force sensors, actuators, controllers, and software components to perform tasks and actions during implant surgery. The system receives commands, controls the robot’s movements and behaviors, and executes the necessary tasks and actions. Presently, research on dental implant robotic systems primarily focuses on the mechanical arm structure and drilling methods.
The majority of dental implant robotic systems directly adopt serial-linked industrial robotic arms based on the successful application of industrial robots with the same robotic arm connection. 59 , 60 , 61 , 62 These studies not only establish implant robot platforms to validate implant accuracy and assess the influence of implant angles, depths, and diameters on initial stability but also simulate chewing processes and prepare natural root-shaped osteotomies based on volume decomposition. Presently, most dental implant robots in research employ a single robotic arm for surgery. Lai et al. 62 indicated that the stability of the handpieces during surgery and real-time feedback of patient movement are crucial factors affecting the accuracy of robot-assisted implant surgery. The former requires physical feedback, while the latter necessitates visual feedback. Hence, they employed a dual-arm robotic system where the main robotic arm was equipped with multi-axis force and torque sensors for performing osteotomies and implant placement. The auxiliary arm consisted of an infrared monocular probe used for visual system positioning to address visual occlusion issues arising from changes in arm angles during surgery.
The robots mentioned above use handpieces to execute osteotomies and implant placement. However, owing to limitations in patient mouth opening, performing osteotomies and placing implants in the posterior region can be challenging. To overcome the spatial constraints during osteotomies in implant surgery, Yuan et al. 63 proposed a robot system based on earlier research which is laser-assisted tooth preparation. This system involves a non-contact ultra-short pulse laser for preparing osteotomies. The preliminary findings confirmed the feasibility of robotically controlling ultra-short pulse lasers for osteotomies, introducing a novel method for a non-contact dental implant robotic system.
Position following of dental implant robotic system
It can be challenging for patients under local anesthesia to remain completely still during robot-assisted dental implant surgery. 52 , 64 , 65 , 66 , 67 Any significant micromovement in the patient’s position can severely affect clinical surgical outcomes, such as surgical efficiency, implant placement accuracy compared to the planned position, and patient safety. Intraoperative movement may necessitate re-registration for certain dental implant robotic systems. In order to guarantee safety and accuracy during surgery, the robot must detect any movement in the patient’s position and promptly adjust the position of the robotic arm in real time. Yakebot uses binocular vision to monitor visual markers placed outside the patient’s mouth and at the end of the robotic arm. This captures motion information and calculates relative position errors. The robot control system utilizes preoperatively planned positions, visual and force feedback, and robot kinematic models to calculate optimal control commands for guiding the robotic arm’s micromovements and tracking the patient’s micromovements during drilling. As the osteotomies are performed to the planned depth, the robotic arm compensates for the patient’s displacement through the position following the function. The Yakebot’s visual system continuously monitors the patient’s head movement in real time and issues control commands every 0.008 s. The robotic arm is capable of following the patient’s movements with a motion servo in just 0.2 s, ensuring precise and timely positioning.
The simulation of surgeons’ tactile sensation in dental implant robotic systems
Robot-assisted dental implant surgery requires the expertise and tactile sense of a surgeon to ensure accurate implantation. Experienced surgeons can perceive bone density through the resistance they feel in their hands and adjust the force magnitude or direction accordingly. This ensures proper drilling along the planned path. However, robotic systems lack perception and control, which may result in a preference for the bone side with lower density. This can lead to inaccurate positioning compared to the planned implant position. 61 , 62 Addressing this challenge, Li et al. 68 established force-deformation compensation curves in the X, Y, and Z directions for the robot’s end-effector based on the visual and force servo systems of the autonomous dental robotic system, Yakebot. Subsequently, a corresponding force-deformation compensation strategy was formulated for this robot, thus proving the effectiveness and accuracy of force and visual servo control through in vitro experiments. The implementation of this mixed control mode, which integrates visual and force servo systems, has improved the robot’s accuracy in implantation and ability to handle complex bone structures. Based on force and visual servo control systems, Chen et al. 69 have also explored the relationship between force sensing and the primary stability of implants placed using the Yakebot autonomous dental robotic system through an in vitro study. A significant correlation was found between Yakebot’s force sensing and the insertion torque of the implants. This correlation conforms to an interpretable mathematical model, which facilitates the predictable initial stability of the implants after placement.
During osteotomies with heat production (which is considered one of the leading causes of bone tissue injury), experienced surgeons could sense possible thermal exposure via their hand feeling. However, with free-handed implant placement surgery, it is challenging to perceive temperature changes during the surgical process and establish an effective temperature prediction model that relies solely on a surgeon’s tactile sense. Zhao et al. 70 , using the Yakebot robotic system, investigated the correlation between drilling-related mechanical data and heat production and established a clinically relevant surrogate for intraosseous temperature measurement using force/torque sensor-captured signals. They also established a real-time temperature prediction model based on real-time force sensor monitoring values. This model aims to effectively prevent the adverse effects of high temperatures on osseointegration, laying the foundation for the dental implant robotic system to autonomously control heat production and prevent bone damage during autonomous robotic implant surgery.
The innovative technologies mentioned above allow dental implant robotic systems to simulate the tactile sensation of a surgeon and even surpass the limitations of human experience. This advancement promises to address issues that free-handed implant placement techniques struggle to resolve. Moreover, this development indicates substantial progress and great potential for implantation.
Clinical research on dental implant robotic systems
Clinical workflow of dental implant robotic systems.
The robotic assistant dental implant surgery consists of three steps: preoperative planning, intraoperative phase, and postoperative phase (Fig. 5 ). For preoperative planning, it is necessary to obtain digital intraoral casts and CBCT data from the patient, which are then imported into preoperative planning software for 3D reconstruction and planning implant placement. For single or multiple tooth gaps using implant robotic systems (except Yakebot), 61 , 62 , 71 , 72 a universal registration device (such as the U-shaped tube) must be worn on the patients’ missing tooth site using a silicone impression material preoperatively to acquire CBCT data for registration. The software performs virtual placement of implant positions based on prosthetic and biological principles of implant surgery, taking into account the bone quality of the edentulous implant site to determine the drilling sequence, insertion depth of each drill, speed, and feed rate. For single or multiple tooth implants performed using Yakebot, there is no need for preoperative CBCT imaging with markers. However, it is necessary to design surgical accessories with registration holes, brackets for attaching visual markers, and devices for assisting mouth opening and suction within the software (Yakebot Technology Co., Ltd., Beijing, China). These accessories are manufactured using 3D printing technology.
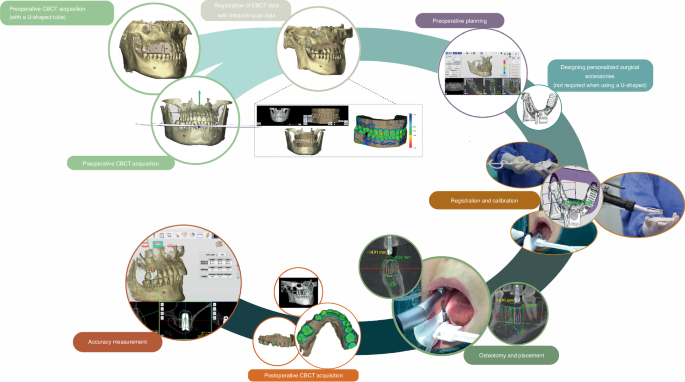
Clinical workflow of robotic-assisted dental implant placement
For the intraoperative phase, the first step is preoperative registration and calibration. For Yakebot, the end-effector marker is mounted to the robotic arm, and the spatial positions are recorded under the optical tracker. The calibration plate with the positioning points is then assembled into the implant handpiece for drill tip calibration. Then, the registration probe is inserted in the registration holes of the jaw positioning plate in turn for spatial registration of the jaw marker and the jaw. Robot-assisted dental implant surgery usually does not require flapped surgery, 73 , 74 , yet bone grafting due to insufficient bone volume in a single edentulous space or cases of complete edentulism requiring alveolar ridge preparation may require elevation of flaps. For full-arch robot-assisted implant surgery, a personalized template with a positioning marker is required and should be fixed with metallic pins for undergoing an intraoperative CBCT examination, thus facilitating the robot and the jaws registration in the visual space and allowing the surgical robot to track the patient’s motion. The safe deployment of a robot from the surgical site is an essential principle for robot-assisted implant surgery. In the case of most robots, such as Yomi, the surgeon needs to hold the handpieces to control and supervise the robot’s movement in real time and stop the robotic arm’s movement in case of any accidents. With Yakebot, the entire surgery is performed under the surgeon’s supervision, and immediate instructions are sent in response to possible emergencies via a foot pedal. Additionally, the recording of the entrance and exit of the patient’s mouth ensures that the instruments would not damage the patient’s surrounding tissues. The postoperative phase aims at postoperative CBCT acquisition and accuracy measurement.
In clinical surgical practice, robots with varying levels of autonomy perform implant surgeries differently. According to the autonomy levels classified by Yang et al. 6 , 8 , 33 for medical robots, commercial dental implant robotic systems (Table 2 ) currently operate at the level of robot assistance or task autonomy.
The robot-assistance dental implant robotic systems provide haptic, 75 visual or combined visual and tactile guidance during dental implant surgery. 46 , 76 , 77 Throughout the procedure, surgeons must maneuver handpieces attached to the robotic guidance arm and apply light force to prepare osteotomies. 62 The robotic arm constrains the 3D space of the drill as defined by the virtual plan, enabling surgeons to move the end of the mechanical arm horizontally or adjust its movement speed. However, during immediate implant placement or full-arch implant surgery, both surgeons and robots may struggle to accurately perceive poor bone quality, which should prompt adjustments at the time of implant placement. This can lead to incorrect final implant positions compared to the planned locations.
The task-autonomous dental implant robotic systems can autonomously perform partial surgical procedures, such as adjusting the position of the handpiece to the planned position and preparing the implant bed at a predetermined speed according to the pre-operative implant plan, and surgeons should send instructions, monitor the robot’s operation, and perform partial interventions as needed. For example, the Remebot 77 , 78 requires surgeons to drag the robotic arm into and out of the mouth during surgery, and the robot automatically performs osteotomies or places implants according to planned positions under the surgeon’s surveillance. The autonomous dental implant robot system, Yakebot, 73 , 79 , 80 can accurately reach the implant site and complete operations such as implant bed preparation and placement during surgery. It can be controlled by the surgeon using foot pedals and automatically stops drilling after reaching the termination position before returning to the initial position. Throughout the entire process, surgeons only need to send commands to the robot using foot pedals.
Clinical performance of robot-assisted implant surgery
Figure 6 shows the results of accuracy in vitro, in vivo, and clinical studies on robot-assisted implant surgery. 20 , 46 , 48 , 55 , 62 , 64 , 67 , 68 , 69 , 70 , 71 , 72 , 75 , 76 , 77 , 78 , 79 , 80 , 81 , 82 , 83 , 84 , 85 , 86 , 87 , 88 , 89 The results suggest that platform and apex deviation values are consistent across different studies. However, there are significant variations in angular deviations among different studies, which may be attributed to differences in the perception and responsiveness to bone quality variances among different robotic systems. Therefore, future development should focus on enhancing the autonomy of implant robots and improving their ability to recognize and respond to complex bone structures.
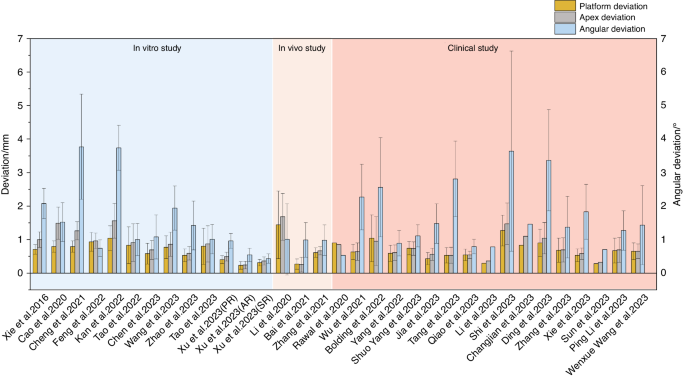
Accuracy reported in studies on robotic-assisted implant placement
Xu et al. 77 conducted a phantom experimental study comparing the implant placement accuracy in three levels of dental implant robotics, namely passive robot (Dcarer, level 1), semi-active robot (Remebot, level 2), and active robot (Yakebot, level 2) (Fig. 7 ). The study found that active robot had the lowest deviations at the platform and apex of the planned and actual implant positions, While the semi-active robot also had the lowest angular deviations. Chen et al. 46 and Jia et al. 79 conducted clinical trials of robotic implant surgery in partially edentulous patients using a semi-active dental implant robotic system (level 1) and an autonomous dental implant robot (level 2). The deviations of the implant platform, apex, and angle were (0.53 ± 0.23) mm/(0.43 ± 0.18) mm, (0.53 ± 0.24) mm/(0.56 ± 0.18) mm and 2.81° ± 1.13°/1.48° ± 0.59°, respectively. These results consistently confirmed that robotic systems can achieve higher implant accuracy than static guidance and that there is no significant correlation between accuracy and implant site (such as anterior or posterior site). The platform and angle deviation of autonomous dental implant robots were smaller than those of semi-active dental implant robotic systems. Li et al. 73 reported the use of the autonomous dental implant robot (level 2) to complete the placement of two adjacent implants with immediate postoperative restoration. The interim prosthesis fabricated prior to implant placement was seated without any adjustment, and no adverse reactions occurred during the operation.
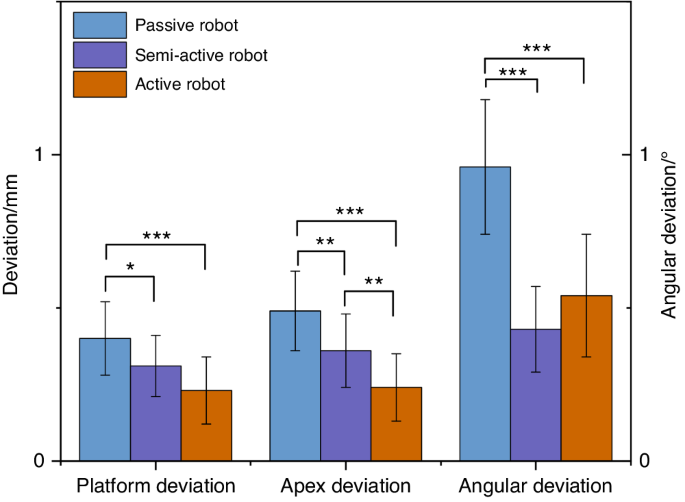
Comparison of accuracy of dental implant robotics with different levels of autonomy (phantom experiments) (* P < 0.05, ** P < 0.01, *** P < 0.001)
Bolding et al., 53 Li et al., 20 Jia et al., 79 and Xie et al. 90 used dental implant robots to conduct clinical trials in full-arch implant surgery with five or six implants placed in each jaw. The deviations of implant platform, apex, and angle are shown in Fig. 8 . The haptic dental implant robot (level 1) used by Bolding et al., 53 achieved more deviations compared to other studies that used semi-active (level 1) or active robots (level 2). As its handpiece must be maneuvered by the surgeon, human errors such as surgeon fatigue may not be avoided. Owing to the parallel common implant placement paths between various implant abutments, prefabricated temporary dentures could be seated smoothly, and some patients wore temporary complete dentures immediately after surgery. These results indicate that robotic systems can accurately locate and perform implant placement during surgery.

Comparison of accuracy in robotic-assisted full-arch implant placement
As there are relatively few studies of implant robots in clinical applications, Tak ́acs et al. 91 conducted a meta-analysis under in vitro conditions with free-handed, static-guided, dynamic navigated, and robotic-assisted implant placements, as shown in Fig. 9 . It was found that, compared to free-handed, static guided and dynamic navigated implant placements, robotic-assisted implant placements have more advantages in terms of accuracy. However, in vitro studies cannot fully simulate the patients’ oral condition and bone quality. Recent clinical studies 89 , 92 , 93 have shown a lower deviation in robotic-assisted implant placements compared to static-guided and dynamic-navigated implant placements. Common reasons for deviations in static-guided and dynamic-navigated implant placements include the following: deflection caused by hand tremors due to dense bone during surgery, surgeons’ experience, and other human factors. Larger clinical studies will be needed in the future to evaluate the differences between robotic and conventional surgical approaches and to provide guidance for the further development and refinement of robotic techniques.
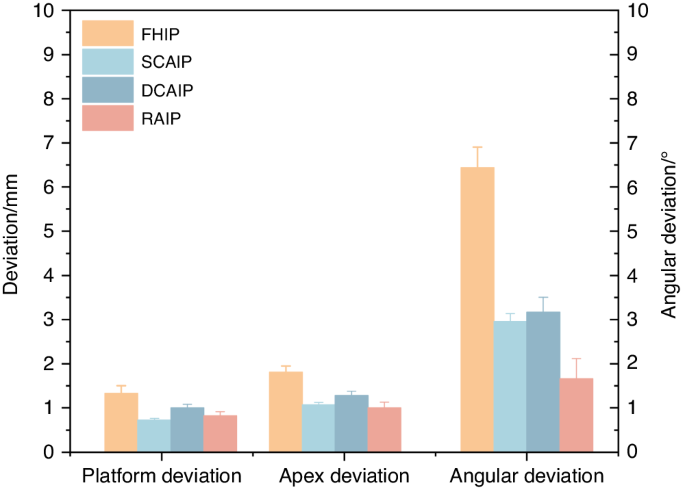
Comparison of accuracy of free-handed, static, dynamic, and robotic-assisted implant placement. (FHIP free-hand implant placement, SCAIP static computer-aided implant placement, DCAIP dynamic computer-aided implant placement, RAIP robot-assisted implant placement)
For the long-term follow-up performance of robotic systems used in dental implant procedures, none of the comparative studies was longer than a year. One 1-year prospective clinical study by Xie et al. 90 showed that the peri-implant tissues after robot-assisted full arch surgery at 1-year visit remained stable. There is little evidence indicating clinical outcomes especially for patient-reported outcomes. A more detailed clinical assessment should be included for further research.
Current issues with dental implant robotic systems
Need for further simplification of robotic surgical procedures.
Although robotic-assisted dental implant surgery can improve accuracy and treatment quality, 94 it involves complex registration, calibration, and verification procedures that prolong the duration of surgery. These tedious processes may introduce new errors, 61 and lower work efficiency, especially in single tooth implant placement 62 that could extend visit times and affect patient satisfaction. 62 Besides, surgeons are required to undergo additional training to familiarize themselves with the robotic system. 87
Need for improved flexibility of dental implant robotic system
During implantation, the drill tips at the end of the robotic arms cannot be tilted, and this can increase the difficulty of using robots in posterior sections with limited occlusal space. 61 , 62 In addition, currently available marker systems require patients to wear additional devices to hold the marker in place. If these markers are contaminated or obstructed by blood, the visual system may not be able to detect them, limiting surgical maneuverability to some extent. During immediate implant placement or in cases of poor bone quality in the implant site, the drill tips may deviate towards the tooth sockets or areas of lower bone density, seriously affecting surgical precision.
Currently, only one study has developed a corresponding force-deformation compensation strategy for robots, 68 but clinical validation is still lacking. Additionally, the dental implant robotic system, along with other dental implant robots developed for prosthetics, endodontics, and orthodontics, is currently single-functional. Multi-functional robots are required for performing various dental treatments.
Difficulties in promoting the use of dental implant robotic system
Despite the enormous potential of robotic systems in the medical field, similar to the development of computer-aided design/computer-aided manufacturing technology, introducing and applying this technology faces multiple challenges in the initial stages. The high cost of robotic equipment may limit its promotion and application in certain regions or medical institutions. Surgeons require specialized technical training before operating robotic systems, which translates to additional training costs and time investment. 95
Prospects in the use of dental implant robotic system
Medical robots possess high-precision sensing and positioning capabilities, which enable precise operations at small scales. They are also equipped with safety mechanisms and stability controls to ensure the safety of medical procedures and reduce risks to patients. As technology evolves, hardware and algorithms are continuously updated, resulting in constant performance improvements. Today, medical robots are widely used in surgery, diagnosis, and rehabilitation. 7 They enable precise and minimally invasive operation, thus reducing patient trauma and pain, shortening hospitalization, and speeding recovery, as well as reducing the need for re-operations and blood transfusions. 96 In addition, medical robots can reduce radiation exposure for both surgeons and patients. By leveraging machine learning and artificial intelligence technologies, robots can provide personalized and intelligent treatment plans and recommendations based on large amounts of data, improving diagnostic efficiency. Robots with remote operation capabilities can enable remote surgeries or consultations across regions, facilitating access to medical services. Moreover, robots can work continuously, ensuring medical quality and consistency while reducing surgeons ’neck and back pain, 97 as well as numbness in the hands and wrists experienced by surgeons. 98 Besides, they also reduce mental and physical stress, improving surgeons’ quality of life and extending their career longevity.
From da Vinci surgical robotic system to dental implant robotic system, these innovative technologies are leading unprecedented changes in the medical field. Dental implant robotic system continuously improves software modules and optimizes operating procedures to become more intelligent, more flexible and easier to learn and use. In the future, more extensive clinical trials will be needed to continuously observe and evaluate the long-term outcomes of robot-assisted implant surgery, especially in multi-center clinical trials. Moreover, measured outcomes must include well-defined clinical outcomes (such as pathophysiology 99 ), technical outcomes (including those derived from robotic kinematic and haptic sensors 100 ), patient-reported outcomes (such as quality-of-life indicators and overall satisfaction with treatment 99 ), and wider outcomes that reflect potential robotic disruption (ergonomic benefits, impacts on accessibility to surgery 100 ) where relevant. In addition, the evaluation of dental implant robots requires the analysis of learning curves. Large prospective cohorts provide the first opportunity to capture real-world learning curves, which can be used to develop training mechanisms that shorten learning curves and minimize any negative impact on patients. 99 , 100
As a pioneering attempt, the dental implant robotic system provides an important exploration and paradigm for the application of another dental robotic system. As technology continues to advance, robotics and artificial intelligence will provide more precise diagnostic and treatment options, more intelligent medical decision support systems, as well as more flexible and precise surgical procedures. These revolutionary technologies will continue to drive advances in medicine and healthcare, opening up new possibilities for future clinical practice.
With novel technology advancements, medical robotics are bringing a new era to medicine. Innovative medical robotics can perform surgical procedures, aid rehabilitation, make diagnoses, achieve robotic laboratory automation and other robots suitable for various functional needs. In the field of dentistry, the most widely utilized robotic system presently is the dental implant robotic system. Implant robotic systems could offer a more flexible approach for the precise planning, and visual and haptic guidance of surgical procedures. Various clinical trials have confirmed the high accuracy of implant robotic-assisted surgery achieved and toward long-term implant success. However, there is still much room for improvement in terms of further simplification, the flexibility of robotic surgical procedures, and systematic education. By leveraging machine learning and artificial intelligence technologies, more precise diagnostic and treatment options, intelligent medical decision support systems, and flexible and precise surgical procedures will be provided for future clinical practice.
Fukuda, T., Dario, P. & Yang, G. Z. Humanoid robotics—history, current state of the art, and challenges. Sci. Robot. 2 , eaar4043 (2017).
Article PubMed Google Scholar
Dong, J. What you should know about the history of robotics. Robot Ind. 1 , 108–114 (2015).
Google Scholar
Standardization, I.O.F. Robots and robotic devices—vocabulary. ISO 8373:2021.
Liu, H. H., Li, L. J., Shi, B., Xu, C. W. & Luo, E. Robotic surgical systems in maxillofacial surgery: a review. Int. J. Oral. Sci. 9 , 63–73 (2017).
Article PubMed PubMed Central Google Scholar
Kwoh, Y. S., Hou, J., Jonckheere, E. A. & Hayati, S. A robot with improved absolute positioning accuracy for CT guided stereotactic brain surgery. IEEE Trans. Biomed. Eng. 35 , 153–160 (1988).
Article CAS PubMed Google Scholar
Troccaz, J., Dagnino, G. & Yang, G. Z. Frontiers of medical robotics: from concept to systems to clinical translation. Annu. Rev. Biomed. Eng. 21 , 193–218 (2019).
Dupont, P. E. et al. A decade retrospective of medical robotics research from 2010 to 2020. Sci. Robot. 6 , eabi8017 (2021).
Yang, G. Z. et al. Medical robotics—regulatory, ethical, and legal considerations for increasing levels of autonomy. Sci. Robot. 2 , eaam8638 (2017).
Yip, M. et al. Artificial intelligence meets medical robotics. Science 381 , 141–146 (2023).
Wang, T. M. et al. Medical Surgical Robotics. China Science Publishing & Media Ltd. http://find.nlc.cn/search/showDocDetails?docId=-9060319075756851951&dataSource=ucs01 (2013).
Liu, Y. et al. Fully automatic AI segmentation of dental implant surgery related tissues based on cone beam computed tomography images. Int. J. Oral Sci. (2024) (Accept for publication).
Alemzadeh, K. & Raabe, D. Prototyping artificial jaws for the robotic dental testing simulator. Proc. Inst. Mech. Eng. Part H 222 , 1209–1220 (2008).
Article CAS Google Scholar
Kazanzides, P. et al. Surgical and interventional robotics: core concepts, technology, and design. IEEE Robot. Autom. Mag. 15 , 122–130 (2008).
Khan, K., Dobbs, T., Swan, M. C., Weinstein, G. S. & Goodacre, T. E. Trans-oral robotic cleft surgery (TORCS) for palate and posterior pharyngeal wall reconstruction: a feasibility study. J. Plast. Reconstr. Aesthet. Surg. 69 , 97–100 (2016).
Nadjmi, N. Transoral robotic cleft palate surgery. Cleft Palate Craniofac. J. 53 , 326–331 (2016).
Vicini, C. et al. Transoral robotic tongue base resection in obstructive sleep apnoea-hypopnoea syndrome: a preliminary report. ORL J. Otorhinolaryngol. Relat. Spec. 72 , 22–27 (2010).
Weinstein, G. S. et al. Transoral robotic surgery alone for oropharyngeal cancer: an analysis of local control. Arch. Otolaryngol. Head. Neck Surg. 138 , 628–634 (2012).
Kayhan, F. T., Kaya, H. & Yazici, Z. M. Transoral robotic surgery for tongue-base adenoid cystic carcinoma. J. Oral. Maxillofac. Surg. 69 , 2904–2908 (2011).
Olivetto, M., Bettoni, J., Testelin, S. & Lefranc, M. Zygomatic implant placement using a robot-assisted flapless protocol: proof of concept. Int. J. Oral. Maxillofac. Surg. 52 , 710–715 (2023).
Li, C. et al. Autonomous robotic surgery for zygomatic implant placement and immediately loaded implant-supported full-arch prosthesis: a preliminary research. Int. J. Implant. Dent. 9 , 12 (2023).
Article CAS PubMed PubMed Central Google Scholar
Saleh, M. A., Hanapiah, F. A. & Hashim, H. Robot applications for autism: a comprehensive review. Disabil. Rehabil. Assist. Technol. 16 , 580–602 (2021).
Chen, X. P. Advancement and challenges of medical robots from an interdisciplinary viewpoint. Chin. Bull. Life Sci. 34 , 965–973 (2022).
Winchester, P. et al. Changes in supraspinal activation patterns following robotic locomotor therapy in motor-incomplete spinal cord injury. Neurorehabil. Neural Repair 19 , 313–324 (2005).
Alashram, A. R., Annino, G. & Padua, E. Robot-assisted gait training in individuals with spinal cord injury: a systematic review for the clinical effectiveness of Lokomat. J. Clin. Neurosci. 91 , 260–269 (2021).
Topping, M. An overview of the development of Handy 1, a rehabilitation robot to assist the severely disabled. Artif. Life Robot. 4 , 188–192 (2000).
Article Google Scholar
Meng, F., Peng, X. Y. & Xu, Y. N. Analysis of and research on the development of lower limb wearable exoskeleton. J. Mech. Transm. 46 , 163–169 (2022).
Ezaki, S. et al. Analysis of gait motion changes by intervention using robot suit hybrid assistive limb (HAL) in myelopathy patients after decompression surgery for ossification of posterior longitudinal ligament. Front. Neurorobot. 15 , 650118 (2021).
Tegally, H., San, J. E., Giandhari, J. & de Oliveira, T. Unlocking the efficiency of genomics laboratories with robotic liquid-handling. BMC Genomics 21 , 729 (2020).
Rupp, N., Peschke, K., Koppl, M., Drissner, D. & Zuchner, T. Establishment of low-cost laboratory automation processes using AutoIt and 4-axis robots. SLAS Technol. 27 , 312–318 (2022).
Wu, Y. H., Fassert, C. & Rigaud, A. S. Designing robots for the elderly: appearance issue and beyond. Arch. Gerontol. Geriatr. 54 , 121–126 (2012).
Liu, Y. et al. Boosting framework via clinical monitoring data to predict the depth of anesthesia. Technol. Health Care 30 , 493–500 (2022).
Yang, G. Z. et al. Combating COVID-19-The role of robotics in managing public health and infectious diseases. Sci. Robot. 5 , eabb5589 (2020).
Gao, A. et al. Progress in robotics for combating infectious diseases. Sci. Robot. 6 , eabf1462 (2021).
Yu, H. et al. Management of systemic risk factors ahead of dental implant therapy: a beard well lathered is half shaved. J. Leukoc. Biol. 110 , 591–604 (2021).
Cheng, L. et al. [A review of peri-implant microbiology]. Hua XI Kou Qiang Yi Xue Za Zhi 37 , 7–12 (2019).
PubMed Google Scholar
Patel, R. & Clarkson, E. Implant surgery update for the general practitioner: dealing with common postimplant surgery complications. Dent. Clin. North Am. 65 , 125–134 (2021).
Herrera, D. et al. Prevention and treatment of peri-implant diseases — the EFP S3 level clinical practice guideline. J. Clin. Periodontol. 50 , 4–76 (2023).
Wu Q., Research on the creation and application of the spatial mapping devices of the dental implant robot system. Fourth Military Medical University. Vol. graduate. (2016).
Ruff, C., Richards, R., Ponniah, A., Witherow, H., Evans, R. & Dunaway, D. Computed maxillofacial image in surgical navigation. Int. J. Comput. Assist. Radiol. Surg. 2 , 412–418 (2007).
Tal, H. & Moses, O. A comparison of panoramic radiography with computed tomography in the planning of implant surgery. Dentomaxillofac. Radiol. 20 , 40–42 (1991).
Tattan, M., Chambrone, L., Gonzalez-Martin, O. & Avila-Ortiz, G. Static computer-aided, partially guided, and free-handed implant placement: a systematic review and meta-analysis of randomized controlled trials. Clin. Oral. Implant. Res. 31 , 889–916 (2020).
Shen, P. et al. Accuracy evaluation of computer-designed surgical guide template in oral implantology. J. Cranio Maxillofac. Surg. 43 , 2189–2194 (2015).
Vercruyssen, M., Fortin, T., Widmann, G., Jacobs, R. & Quirynen, M. Different techniques of static/dynamic guided implant surgery: modalities and indications. Periodontology 2000 66 , 214–227 (2014).
Zhao Y. Clinical study of an autonomous dental implant robot. In: 2021 Compendium of Papers from the Sixth National Oral and Maxillofacial Prosthodontics Annual Meeting of the Oral and Maxillofacial Prosthodontics Committee of the Chinese Dental Association . 7-8 https://doi.org/10.26914/c.cnkihy.2021.063176 (2021).
Kivovics, M., Takacs, A., Penzes, D., Nemeth, O. & Mijiritsky, E. Accuracy of dental implant placement using augmented reality-based navigation, static computer assisted implant surgery, and the free-hand method: an in vitro study. J. Dent. 119 , 104070 (2022).
Chen, W. et al. Accuracy of dental implant placement with a robotic system in partially edentulous patients: a prospective, single-arm clinical trial. Clin. Oral. Implant. Res. 34 , 707–718 (2023).
Gwangho, K., Hojin, S., Sungbeen, I., Dongwan, K. & Sanghwa, J. A study on simulator of human-robot cooperative manipulator for dental implant surgery. 2009 IEEE International Symposium on Industrial Electronics, Seoul, Korea (South) . 2159-2164 https://doi.org/10.1109/ISIE.2009.5222561 (2009).
Alqutaibi, A. Y., Hamadallah, H. H., Abu, Z. B., Aloufi, A. M. & Tarawah, R. A. Applications of robots in implant dentistry: a scoping review. J. Prosthet. Dent . 11:S0022-3913(23)00770-9. Epub ahead of print. https://doi.org/10.1016/j.prosdent.2023.11.019 (2023).
Dutreuil, J. G. F. L. Computer Assisted Dental Implantology: A New Method and a Clinical Validation. In: Niessen, W.J., Viergever, M.A. (eds) Medical Image Computing and Computer-Assisted Intervention – MICCAI 2001. MICCAI 2001. 2208 https://doi.org/10.1007/3-540-45468-3_46 (2001).
R. Boesecke, J. B. J. R. Robot Assistant for Dental Implantology . (Springer: Berlin, Heidelberg, 2001). .
Sun, X. et al. Automated dental implantation using image-guided robotics: registration results. Int. J. Comput. Assist. Radiol. Surg. 6 , 627–634 (2011).
Sun, X., Yoon, Y., Li, J. & Mckenzie, F. D. Automated image-guided surgery for common and complex dental implants. J. Med. Eng. Technol. 38 , 251–259 (2014).
Bolding, S. L. & Reebye, U. N. Accuracy of haptic robotic guidance of dental implant surgery for completely edentulous arches. J. Prosthet. Dent. 128 , 639–647 (2022).
Zhou, G. et al. Intraoperative localization of small pulmonary nodules to assist surgical resection: a novel approach using a surgical navigation puncture robot system. Thorac. Cancer 11 , 72–81 (2020).
K, Y. et al. Stereo vision based robot navigation system using modulated potential field for implant surgery. IEEE International Conference on Industrial Technology 493–498 https://doi.org/10.1109/ICIT.2015.7125147 (2015).
S, Y., A, M. P. & Y, V. D. R., Automation of end effector guidance of robotic arm for dental implantation using computer vision. IEEE Distributed Computing, VLSI, Electrical Circuits and Robotics 84–89. https://doi.org/10.1109/DISCOVER.2016.7806263 (2016).
Yan, B. et al. Optics-guided Robotic System for Dental Implant Surgery. Chin. J. Mech. Eng. 35 , 55 (2022).
Xie R. The study on accurary of the Dental Implantology Robotic System. Fourth Military Medical University . Vol. graduate. (2016).
Wilmes, B. & Drescher, D. Impact of insertion depth and predrilling diameter on primary stability of orthodontic mini-implants. Angle Orthod. 79 , 609–614 (2009).
Wilmes, B., Su, Y. Y. & Drescher, D. Insertion angle impact on primary stability of orthodontic mini-implants. Angle Orthod. 78 , 1065–1070 (2008).
Shi, J. Y. et al. Improved positional accuracy of dental implant placement using a haptic and machine-vision-controlled collaborative surgery robot: a pilot randomized controlled trial. J. Clin. Periodontol. 51 , 24–32 (2024).
Qiao, S. C., Wu, X. Y., Shi, J. Y., Tonetti, M. S. & Lai, H. C. Accuracy and safety of a haptic operated and machine vision controlled collaborative robot for dental implant placement: a translational study. Clin. Oral. Implant. Res. 34 , 839–849 (2023).
Yuan, F. S. et al. Preliminary study on the automatic preparation of dental implant socket controlled by micro-robot. Zhonghua Kou Qiang Yi Xue Za Zhi 53 , 524–528 (2018).
CAS PubMed Google Scholar
Kan, T. S. et al. Evaluation of a custom-designed human-robot collaboration control system for dental implant robot. Int. J. Med. Robot. Comput. Assist. Surg. 18 , e2346 (2022).
Cheng, K. J. et al. Accuracy of dental implant surgery with robotic position feedback and registration algorithm: an in-vitro study. Comput. Biol. Med. 129 , 104153 (2021).
Feng, Y. et al. An image-guided hybrid robot system for dental implant surgery. Int. J. Comput. Assist. Radiol. Surg. 17 , 15–26 (2022).
Tao, B. et al. The accuracy of a novel image-guided hybrid robotic system for dental implant placement: an in vitro study. Int. J. Med. Robot. Comput. Assist. Surg. 19 , e2452 (2023).
Li Z. W. The study on accuracy of the Dental Implantology Robotic System. Air Force Medical University Vol. Graduate. (2021).
Chen, D., Chen, J., Wu, X., Chen, Z. & Liu, Q. Prediction of primary stability via the force feedback of an autonomous dental implant robot. J. Prosthet. Dent . S0022-3913(23)00755-2. Epub ahead of print. https://doi.org/10.1016/j.prosdent.2023.11.008 (2023).
Zhao, R. et al. Correlation between intraosseous thermal change and drilling impulse data during osteotomy within autonomous dental implant robotic system: an in vitro study. Clin. Oral Implant. Res. 35 , 258–267 (2023).
Yang, S. et al. Accuracy of autonomous robotic surgery for single-tooth implant placement: a case series. J. Dent. 132 , 104451 (2023).
Rawal, S., Tillery, D. J. & Brewer, P. Robotic-assisted prosthetically driven planning and immediate placement of a dental implant. Compend. Contin. Educ. Dent. 41 , 26–30 (2020).
Li, Z., Xie, R., Bai, S. & Zhao, Y. Implant placement with an autonomous dental implant robot: a clinical report. J. Prosthet. Dent . S0022-3913(23)00124-5. Epub ahead of print. https://doi.org/10.1016/j.prosdent.2023.02.014 (2023).
Talib, H. S., Wilkins, G. N. & Turkyilmaz, I. Flapless dental implant placement using a recently developed haptic robotic system. Br. J. Oral. Maxillofac. Surg. 60 , 1273–1275 (2022).
Ali, M. Flapless dental implant surgery enabled by haptic robotic guidance: a case report. Clin. Implant Dent. Relat. Res . Epub ahead of print. https://doi.org/10.1111/cid.13279 (2023).
Chen, J. et al. Comparison the accuracy of a novel implant robot surgery and dynamic navigation system in dental implant surgery: an in vitro pilot study. BMC Oral. Health 23 , 179 (2023).
Xu, Z. et al. Accuracy and efficiency of robotic dental implant surgery with different human-robot interactions: an in vitro study. J. Dent. 137 , 104642 (2023).
Yang, S., Chen, J., Li, A., Li, P. & Xu, S. Autonomous robotic surgery for immediately loaded implant-supported maxillary full-arch prosthesis: a case report. J. Clin. Med. 11 , 6594 (2022).
Jia, S., Wang, G., Zhao, Y. & Wang, X. Accuracy of an autonomous dental implant robotic system versus static guide-assisted implant surgery: a retrospective clinical study. J. Prosthet. Dent . S0022-3913(23)00284-6. Epub ahead of print. https://doi.org/10.1016/j.prosdent.2023.04.027 (2023).
Bai, S. Z. et al. Animal experiment on the accuracy of the Autonomous Dental Implant Robotic System. Zhonghua Kou Qiang Yi Xue Za Zhi 56 , 170–174 (2021).
Zhao, Y. et al. Effect of the number and distribution of fiducial markers on the accuracy of robot-guided implant surgery in edentulous mandibular arches: an in vitro study. J. Dent. 134 , 104529 (2023).
Mozer, P. S. Accuracy and deviation analysis of static and robotic guided implant surgery: a case study. Int. J. Oral. Maxillofac. Implants 35 , e86–e90 (2020).
Chen, J. et al. Accuracy of immediate dental implant placement with task-autonomous robotic system and navigation system: an in vitro study. Clin. Oral Implant. Res . Epub ahead of print. https://doi.org/10.1111/clr.14104 (2023).
Cao, Z. et al. Pilot study of a surgical robot system for zygomatic implant placement. Med. Eng. Phys. 75 , 72–78 (2020).
Zhang, K., Yu, M. L., C, C. & Xu, B. H. Preliminary research on the accuracy of implant surgery assisted by implant surgery robot. China Med. Device Inf. 27 , 25–28 (2021).
CAS Google Scholar
Tao, B. et al. Accuracy of dental implant surgery using dynamic navigation and robotic systems: an in vitro study. J. Dent. 123 , 104170 (2022).
Ding, Y. et al. Accuracy of a novel semi-autonomous robotic-assisted surgery system for single implant placement: a case series. J. Dent. 139 , 104766 (2023).
Li, P. et al. Accuracy of autonomous robotic surgery for dental implant placement in fully edentulous patients: a retrospective case series study. Clin. Oral. Implant. Res. 34 , 1428–1437 (2023).
Wang, W. et al. Accuracy of the Yakebot dental implant robotic system versus fully guided static computer-assisted implant surgery template in edentulous jaw implantation: a preliminary clinical study. Clin. Implant Dent. Relat. Res . https://doi.org/10.1111/cid.13278 . Epub ahead of print (2023).
Xie, R. et al. Clinical evaluation of autonomous robotic-assisted full-arch implant surgery: a 1-year prospective clinical study. Clin. Oral Implant. Res . Epub ahead of print. https://doi.org/10.1111/clr.14243 (2024).
Takacs, A. et al. Advancing accuracy in guided implant placement: a comprehensive meta-analysis: meta-analysis evaluation of the accuracy of available implant placement methods. J. Dent. 139 , 104748 (2023).
He, J. et al. In vitro and in vivo accuracy of autonomous robotic vs. fully guided static computer-assisted implant surgery. Clin. Implant Dent. Relat. Res. https://doi.org/10.1111/cid.13302 (2024). Epub ahead of print.
Zhang, S. et al. Accuracy of implant placement via dynamic navigation and autonomous robotic computer-assisted implant surgery methods: a retrospective study. Clin. Oral. Implant. Res. 35 , 220–229 (2024).
Shi, B. & Huang, H. Computational technology for nasal cartilage-related clinical research and application. Int. J. Oral. Sci. 12 , 21 (2020).
Zhou, L., Teng, W., Li, X. & Su, Y. Accuracy of an optical robotic computer-aided implant system and the trueness of virtual techniques for measuring robot accuracy evaluated with a coordinate measuring machine in vitro. J. Prosthet. Dent . 11:S0022-3913(23)00751-5. Epub ahead of print. https://doi.org/10.1016/j.prosdent.2023.11.004 (2023).
Forsmark, A. et al. Health economic analysis of open and robot-assisted laparoscopic surgery for prostate cancer within the prospective multicentre LAPPRO trial. Eur. Urol. 74 , 816–824 (2018).
Rokhshad, R., Keyhan, S. O. & Yousefi, P. Artificial intelligence applications and ethical challenges in oral and maxillo-facial cosmetic surgery: a narrative review. Maxillofac. Plast. Reconstr. Surg. 45 , 14 (2023).
Gofrit, O. N. et al. Surgeons’ perceptions and injuries during and after urologic laparoscopic surgery. Urology 71 , 404–407 (2008).
Tonetti, M. S. et al. Relevant domains, core outcome sets and measurements for implant dentistry clinical trials: the Implant Dentistry Core Outcome Set and Measurement (ID-COSM) international consensus report. J. Clin. Periodontol. 50 , 5–21 (2023).
Marcus, H. J. et al. The IDEAL framework for surgical robotics: development, comparative evaluation and long-term monitoring. Nat. Med. 30 , 61–75 (2024).
Sun, Z. J. & Tian, Z. M. Advances in neurosurgical surgical robotics. Chin. J. Minimally Invasive Neurosurg 5 , 238–240 (2008).
Abdul-Muhsin, H. P. V. History of Robotic Surgery. In: (eds Kim, K.) Robotics in General Surgery . (Springer, New York, NY, 2014).
Ewing, D. R., Pigazzi, A., Wang, Y. & Ballantyne, G. H. Robots in the operating room–the history. Semin. Laparosc. Surg. 11 , 63–71 (2004).
Leal, G. T. & Campos, C. O. 30 Years of robotic surgery. World J. Surg. 40 , 2550–2557 (2016).
Falcone, T., Goldberg, J., Garcia-Ruiz, A., Margossian, H. & Stevens, L. Full robotic assistance for laparoscopic tubal anastomosis: a case report. J. Laparoendosc. Adv. Surg. Tech. 9 , 107–113 (1999).
Maeso, S. et al. Efficacy of the Da Vinci surgical system in abdominal surgery compared with that of laparoscopy: a systematic review and meta-analysis. Ann. Surg. 252 , 254–262 (2010).
M, J. et al. The hands-on orthopaedic robot “acrobot”: Early clinical trials of total knee replacement surgery. IEEE Trans. Robot. Autom. 19 , 902–911 (2003).
Schweikard, A., Shiomi, H. & Adler, J. Respiration tracking in radiosurgery. Med. Phys. 31 , 2738–2741 (2004).
Lieberman, I. H. et al. Bone-mounted miniature robotic guidance for pedicle screw and translaminar facet screw placement: Part I—technical development and a test case result. Neurosurgery 59 , 641–650 (2006).
Reddy, V. Y. et al. View-synchronized robotic image-guided therapy for atrial fibrillation ablation: experimental validation and clinical feasibility. Circulation 115 , 2705–2714 (2007).
Subramanian, P., Wainwright, T. W., Bahadori, S. & Middleton, R. G. A review of the evolution of robotic-assisted total hip arthroplasty. Hip Int. 29 , 232–238 (2019).
S, V., G, P. H., J, F. M., J, A. L. & P, C. ViKY robotic scope holder: initial clinical experience and preliminary results using instrument tracking. IEEE/ASME Trans. Mechatron. 15 , 879–886 (2010).
Zhao, R. F., Li, Z. W. & Bai, S. Z. Application of surgical robots in stomatology. Chin. J. Robot. Surg. 3 , 351–366 (2022).
Riga, C. V., Bicknell, C. D., Rolls, A., Cheshire, N. J. & Hamady, M. S. Robot-assisted fenestrated endovascular aneurysm repair (FEVAR) using the Magellan system. J. Vasc. Interv. Radiol. 24 , 191–196 (2013).
Herry, Y. et al. Improved joint-line restitution in unicompartmental knee arthroplasty using a robotic-assisted surgical technique. Int. Orthop. 41 , 2265–2271 (2017).
Wu, Q. & Zhao, Y. M. Application of robotics in stomatology. Int. J. Comput. Dent. 45 , 615–620 (2018).
Lang, S. et al. A european multicenter study evaluating the flex robotic system in transoral robotic surgery. Laryngoscope 127 , 391–395 (2017).
Download references
This work was supported by the National Natural Science Foundation of China [grant number 81970987].
Author information
These authors contributed equally: Chen Liu, Yuchen Liu
Authors and Affiliations
State Key Laboratory of Oral & Maxillofacial Reconstruction and Regeneration, Xi’an, China
Chen Liu, Yuchen Liu, Rui Xie, Zhiwen Li, Shizhu Bai & Yimin Zhao
National Clinical Research Center for Oral Diseases, Xi’an, China
Shaanxi Key Laboratory of Stomatology, Xi’an, China
Digital Center, School of Stomatology, The Fourth Military Medical University, Xi’an, China
You can also search for this author in PubMed Google Scholar
Contributions
Conceptualization, Bai S.Z., and Zhao Y.M.; writing—original draft preparation, Liu C and Liu Y.C.; writing—review and editing, Xie R and Li Z.W., Bai S.Z., and Zhao Y.M. All authors have read and agreed to the published version of the paper.
Corresponding authors
Correspondence to Shizhu Bai or Yimin Zhao .
Ethics declarations
Competing interests.
The authors declare no competing interests.
Rights and permissions
Open Access This article is licensed under a Creative Commons Attribution 4.0 International License, which permits use, sharing, adaptation, distribution and reproduction in any medium or format, as long as you give appropriate credit to the original author(s) and the source, provide a link to the Creative Commons licence, and indicate if changes were made. The images or other third party material in this article are included in the article’s Creative Commons licence, unless indicated otherwise in a credit line to the material. If material is not included in the article’s Creative Commons licence and your intended use is not permitted by statutory regulation or exceeds the permitted use, you will need to obtain permission directly from the copyright holder. To view a copy of this licence, visit http://creativecommons.org/licenses/by/4.0/ .
Reprints and permissions
About this article
Cite this article.
Liu, C., Liu, Y., Xie, R. et al. The evolution of robotics: research and application progress of dental implant robotic systems. Int J Oral Sci 16 , 28 (2024). https://doi.org/10.1038/s41368-024-00296-x
Download citation
Received : 15 January 2024
Revised : 11 March 2024
Accepted : 13 March 2024
Published : 08 April 2024
DOI : https://doi.org/10.1038/s41368-024-00296-x
Share this article
Anyone you share the following link with will be able to read this content:
Sorry, a shareable link is not currently available for this article.
Provided by the Springer Nature SharedIt content-sharing initiative
Quick links
- Explore articles by subject
- Guide to authors
- Editorial policies

- Share full article
Advertisement
Supported by
Despite Setback, Neuralink’s First Brain-Implant Patient Stays Upbeat
Elon Musk’s first human experiment with a computerized brain device developed significant flaws, but the subject, who is paralyzed, has few regrets.

By Christina Jewett
Just four months ago, Noland Arbaugh had a circle of bone removed from his skull and hair-thin sensor tentacles slipped into his brain. A computer about the size of a small stack of quarters was placed on top and the hole was sealed.
Paralyzed below the neck, Mr. Arbaugh is the first patient to take part in the clinical trial of humans testing Elon Musk’s Neuralink device, and his early progress was greeted with excitement.
Working with engineers, Mr. Arbaugh, 30, trained computer programs to translate the firing of neurons in his brain into the act of moving a cursor up, down and around. His command of the cursor was soon so agile that he could challenge his stepfather at Mario Kart and play an empire-building video game late into the night.
But as weeks passed, about 85 percent of the device’s tendrils slipped out of his brain. Neuralink’s staff had to retool the system to allow him to regain command of the cursor. Though he needed to learn a new method to click on something, he can still skate the cursor across the screen.
Neuralink advised him against a surgery to replace the threads, he said, adding that the situation had stabilized.
The setback became public earlier this month. And although the diminished activity was initially difficult and disappointing, Mr. Arbaugh said it had been worth it for Neuralink to move forward in a tech-medical field aimed at helping people regain their speech, sight or movement.
“I just want to bring everyone along this journey with me,” he said. “I want to show everyone how amazing this is. And it’s just been so rewarding. So I’m really excited to keep going.”
From a small desert town in Arizona, Mr. Arbaugh has emerged as an enthusiastic spokesman for Neuralink, one of at least five companies leveraging decades of academic research to engineer a device that can help restore function in people with disabilities or degenerative diseases.
While Mr. Musk’s pitches have centered on sci-fi ambitions like telepathy for high-tech consumers, Mr. Arbaugh’s experience shows the potential for advancement in one medical realm where federal authorities will allow such risky research.
Neuralink announced this week in news reports that it had received permission from the Food and Drug Administration to continue testing implants in additional patients. The company has not offered much detail on the unexpected flaw, and did not respond to requests for comment.
Mr. Arbaugh has been paralyzed since a swimming accident in the lush hills of Northeastern Pennsylvania, where he worked after college as a camp counselor. Lunging into waist-deep water in a lake with a group of friends, he sank to the bottom.
“I was facedown in the water and I just thought, well, I can’t move. So what do I do? I guess nothing,” Mr. Arbaugh said. “So I took a big drink and passed out.”
Mr. Arbaugh became paralyzed from the fourth vertebra in his neck down.
Adjusting to life as a person with quadriplegia was far from the future he had envisioned. As a young person growing up in Yuma, Ariz., Mr. Arbaugh had pursued all his community had to offer. If he wasn’t playing soccer, football or golf, he was at an Academic Decathlon competition or a chess tournament. Among the first in his family to go to college, he enrolled at Texas A&M University, where he admittedly smoked too much marijuana, spent a slightly aimless semester in Australia and skipped too many classes to graduate as a senior.
In the years after the accident, he tried to adjust to an array of devices meant to help people who are paralyzed. Most failed to work effectively for long periods, though Siri on his iPad emerged as his most reliable assistant, enabling him to call and text his friends.
Last year, a friend, Greg Bain, told him about Neuralink and urged him to apply for the company’s first trial in humans.
Mr. Arbaugh said he did not have strong feelings about Mr. Musk, but felt that he drove progress and that “things he touched turned to gold.”
After the implant was embedded in late January, he began working long days with Neuralink staff to link the neuronal patterns picked up in his brain to the actions he intended to take. He found the work tedious and repetitive, but rewarding.
Once the training was complete, engineers gave him control of the cursor on a computer. “I was like, once you guys take these restraints off me, I’m just gonna fly,” Mr. Arbaugh recalled.
On his first day flying solo, Mr. Arbaugh beat a 2017 world record in the field for speed and precision in cursor control. “It was very, very cool,” Mr. Arbaugh said.
The long days of training computer models with Neuralink staff at his side have now been reduced to remote work in four-hour time blocks, Mr. Arbaugh said. The team continues to work on tasks like spelling words, as he envisions making sign language letters or writing on a chalk board.
But the Neuralink device continued to lose its connection, the tendrils gradually sliding out of the tissue of his brain and presumably resting in the fluid that surrounds it.
When only about 15 percent of the threads remained in place, Mr. Arbaugh lost command of the cursor altogether. Engineers recalibrated the computer programs to perform most tasks he had been able to do before. Because he can no longer get the system to do mouse clicks, he’s using a new tool that allows him to click by hovering a cursor over the item he intends to select.
The flawed implant underscores the concerns of some experts in the brain-computer-interface field. The small, round device implanted in the skull is supposed to keep the thin tendrils of electrodes in place. But like a finger in a pie that’s wobbling about, the threads can pull out.
Mr. Arbaugh said his brain moved more than engineers had expected, and have revised the surgical plan to implant the threads deeper in the next patient’s brain.
Neuralink is vetting applications from others interested in taking part in trials. Their expenses, such as travel, are covered by the company , according to Neuralink.
This first Neuralink experiment also highlights how complicated the mechanics of the connection between the brain and a device are.
Lee Miller, a professor of neuroscience and rehabilitative medicine at Northwestern University, described the difficulties of working with the brain. It is bathed in salt water, moves around as the head swivels and bobs, and it is equipped with immune defenses meant to wall off invaders. Researchers have observed the brain forming scar tissue around sensors and even rejecting an entire sensing unit that used a grid of tiny needles.
Cristin Welle, a University of Colorado neurophysiologist who started the neural interfaces program at the Food and Drug Administration, which approves medical devices like the implants, said the first Neuralink case suggested that the company still faced hurdles in developing a durable device.
If the threads were implanted deeper, they could still ease out and leave fibers rubbing on the surface of the brain, possibly increasing the amount of scarring — and signal loss — in the area, she said.
“It’s hard to know if that would work,” Dr. Welle said. “It may be the case that a fully flexible device may not be a long-term solution.”
Mr. Arbaugh said his team had expected his brain to form scar tissue around the threads at the base of the brain — which they believed would help hold them in place. He said he will have the option to leave the study after a year, but expected to keep working with the company longer. Neuralink has said the initial study will take about six years to complete.
Other leading commercial companies have taken different approaches.
Synchron, based in Brooklyn, has avoided the delicate tissue of the brain by going through a vessel to implant a tiny metal tube near the motor cortex of the brain. Yet the device does not pick up as much subtle neural activity as others that penetrate brain tissue, according to researchers in the field. It registers louder signals, so to speak, like the intent to select an option from an on-screen menu. The company has human trials underway .
Precision Neuroscience, based in Manhattan, has implanted a flexible strip equipped with sensors on the surface of people’s brains and is reviewing the data it is gleaning from patients with the strip placed temporarily, Michael Mager, the company’s chief executive, said.
Researchers have been studying brain-computer devices for decades. The standard had been a grid of 96 pins, called the Utah Array, that rests on the top of the brain and picks up activity up to 1.5 millimeters below the surface. It tends to be linked through a wire in the skull to a small box mounted on the head during continuing human trials . The hole in the skull that lets the wire through is prone to infection, though, and Blackrock Neurotech in Salt Lake City is working on a fully implantable upgrade.
Paradromics, which also uses a device with a grid based on the Utah Array, is testing its implantable device in sheep and expects to test it in humans in about a year, according to Matt Angle, the company’s chief executive.
All of the work is closely regulated by the F.D.A., which weighs the risks and the benefits for procedures and is expected to first consider use of these devices in people with major disabilities or degenerative diseases. (The agency would not comment specifically on Neuralink, but said it requires routine reports on expected and unexpected events in such trials.)
Beyond that, researchers are divided over the prospect of widespread use by people with no disability, who might want an implant to communicate without speech or to download a language, as Mr. Musk has mused. Some researchers predict availability for general consumers in decades. Others argue that will never be authorized for activities like web surfing in the shower, given the infection risk of repeated brain surgeries over a lifetime.
Mr. Angle, of Paradromics, said he could envision a progression from usage in people with no ability to speak or walk to those with serious mental health problems that have resisted treatment.
From there, he said, consumer use could be a decade away. After all, he said, the idea of Botox went from preposterous to mainstream in about as much time.
“If 100 years ago you said, ‘Hey, rich people are going to inject botulism in their face,’ that sounds totally nuts,” he said. “Once you understand the risks, and people can make informed decisions, then it becomes reasonable.”
Mr. Arbaugh hopes to see the technology deployed first to restore function in those who lost it.
“And then it can go to enabling people to enhance their capabilities,” he said, adding, “As long as we don’t give up our humanity along the way.”
Christina Jewett covers the Food and Drug Administration, which means keeping a close eye on drugs, medical devices, food safety and tobacco policy. More about Christina Jewett
The World of Elon Musk
The billionaire’s portfolio includes the world’s most valuable automaker, an innovative rocket company and plenty of drama..
Town Halls: X will host live video town halls with Donald Trump and Robert F. Kennedy Jr. in the run-up to the U.S. presidential election, a major push into politics by the social media platform.
SpaceX: At a time when the U.S. government is concerned about its reliance on a mercurial billionaire for access to space, new competitors say Elon Musk’s company is using tactics intended to squash them .
X: Musk has increasingly been using his social media platform to criticize President Biden for his health and immigration policies, according to a New York Times analysis.
Neuralink: Musk’s first human experiment with a computerized brain device developed significant flaws, but the subject, who is paralyzed, has few regrets .
Wooing World Leaders: Musk has fostered relationships with a constellation of right-wing heads of state — including Argentina’s Javier Milei and India’s Narendra Modi — to push his own politics and expand his business empire .
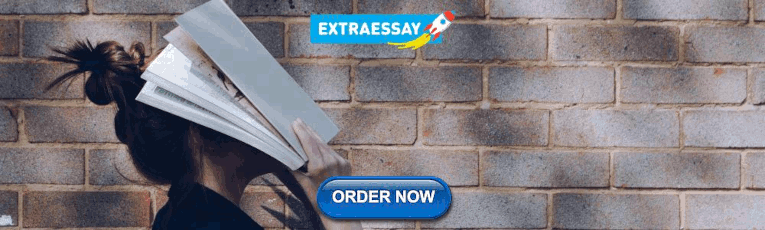
IMAGES
VIDEO
COMMENTS
The papers were published within the last 5 years (from 2013 to 2017) and included dental implants that were less than 8 mm. The period of follow-up ranged from 1 to 5 years. The length of the dental implant varied from 4 to 6.6 mm, and a comparison with long or standard dental implants also varied with and without bone grafts.
Explores the history of dental implants. Reviews the evidence for tissue- and bone-level implants. Discusses the role of the prosthetic connection on bone levels including platform switching.
Dental implants are a common treatment for the loss of teeth. This paper summarizes current knowledge on. implant surfaces, immediate loading versus conventional loading, short implants, sinus ...
Dental implants are becoming a common practice in the field of dentistry. There has been extensive research in this field, and efforts are being made to improve quality while minimizing costs.A comprehensive study was conducted on diverse dental implants, their design procedures, manufacturing methods using additive manufacturing, and materials as well as future developments.
Efficacy and safety of using antibiotics to prevent post-operative complications in oral implant treatment: evidence-based review. Javed Ikram. Rawand Shado. Haidar Hassan. Reviews Open Access 31 ...
Lorwicheanrung J, Mahardawi B, Arunjaroensuk S, Kaboosaya B, Mattheos N, Pimkhaokham A. 2023. The accuracy of implant placement using a combination of static and dynamic computer-assisted implant surgery in fully edentulous arches: a prospective controlled clinical study. Clin Oral Implants Res [epub ahead of print 26 Sep 2023].
Surgical research in implant dentistry. British Dental Journal 235 , 927 ( 2023) Cite this article. Editor: Michel M. Dard; 2023; Springer Cham; £111.50 (eBook); pp. 453; ISBN: 978-3-031-37234-6 ...
Clinical Oral Implants Research is a dentistry and oral surgery journal publishing papers on scientific progress in the field of implant dentistry. Abstract Objectives This scoping review aimed to identify the available evidence in the use of 3D printing technology in dental implantology.
Clinical Oral Implants Research is a dentistry and oral surgery journal publishing papers on scientific progress in the field of implant dentistry and its related areas including digital dentistry. Our articles are relevant to clinicians, teachers and researchers interested how this can benefit patients in need of oral implants.
Clinical Implant Dentistry and Related Research aims to advance the scientific and technical developments related to dental implants and related subjects. Our journal is an ideal resource for clinicians, researchers, teachers and students using and/or studying osseointegrated implants in the oral and maxillofacial areas.
Journal of Dental Implants. 13(1):1, Jan-Jun 2023. Favorite; PDF; Permissions Open. ORIGINAL ARTICLE Estimation of positional accuracy in all on four implants using various splinting materials: An in vitro study. Thakur, Jhalak; Parlani, Swapnil Journal of Dental Implants. 13(1):2-11 ...
This paper outlines the current status and mechanism for identifying dental implants, with emphasis on future direction and updated technology, and covers the existing factors influencing the identification of implant systems. A search was performed on the current methods of identifying dental implants between January 2000 through Feb 2020 using online databases for articles published in English.
The goal of researchers has been to replace normal teeth function and prevent the peri-implantitis issues using dental implants made of novel materials that trigger the osseointegration processes. Figure 2 shows a clear increase implant research from the Web of Science database. Data were obtained using filters with the keyword "peri ...
The goal of the current publication is to provide a comprehensive literature review on the topic of dental implant materials. The following paper focuses on conventional titanium implants and more ...
This review aims to identify and summarise the findings of published qualitative studies relating to patients' experiences of dental implant surgery, by means of textual narrative synthesis. A comprehensive two-stage electronic and manual search of the literature identified relevant qualitative studies up to January 2020. Included primary studies (n = 15) used qualitative research methods ...
In recent years, 3D-printing technology to fabricate dental implants has garnered widespread attention due to its patient-specific customizability and cost-effectiveness. This preclinical animal ...
to 44 have lost at least one permanent tooth to an accident, gum disease, a failed root canal or tooth decay. Furt hermore, by age 74, 26% of adults have lost all of their permanent. teeth ...
Feature papers represent the most advanced research with significant potential for high impact in the field. A Feature Paper should be a substantial original Article that involves several techniques or approaches, provides an outlook for future research directions and describes possible research applications. ... After placing the dental ...
"The dream of many research groups and companies is to implant something into the body that over the long term the body will not see, and the device can provide therapeutic or diagnostic functionality. Now we have such an 'invisibility cloak,' and this is very general: There's no need for a drug, no need for a special polymer," says ...
Here's how dental implant surgery works. For those choosing dental implants for damaged teeth, the first step is to remove the compromised tooth/teeth, root and all. This may require time to heal, before the patient can have dental implants placed. Missing teeth often lead to bone loss, which may preclude some from receiving dental implants.
These papers were screened and many clinical studies were identified, relating to either studies of just virtual implant planning accuracy or studies comparing the accuracy of different types of ...
A Feature Paper should be a substantial original Article that involves several techniques or approaches, provides an outlook for future research directions and describes possible research applications. Feature papers are submitted upon individual invitation or recommendation by the scientific editors and must receive positive feedback from the ...
A total of 2875 clinical studies published between. 2001 and 2012 met the inclusion criteria and were subjected to statistical analysis. Hot topics in. dental implant literature included immediate ...
Objectives Current evidence supports the benefits of cochlear implants (CIs) in children with hearing loss, including those with auditory neuropathy spectrum disorder (ANSD). However, there is limited evidence regarding factors that hold predictive value for intervention outcomes. Design This retrospective case-control study consisted of 66 children with CIs, including 22 with ANSD and 44 with ...
This article reviewed the history of medical robot development and seminal research papers about current research progress. Taking the autonomous dental implant robotic system as an example, the ...
The flawed implant underscores the concerns of some experts in the brain-computer-interface field. The small, round device implanted in the skull is supposed to keep the thin tendrils of ...