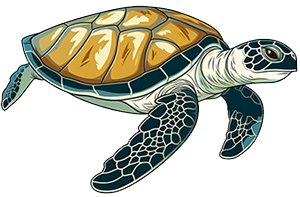
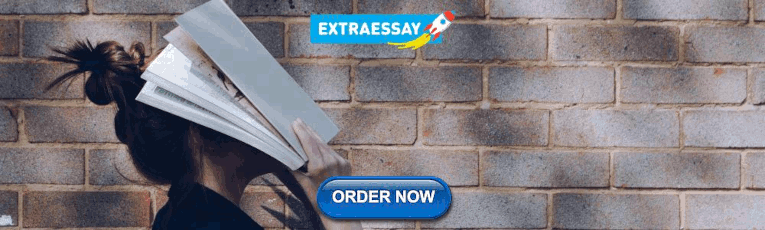
The Biology Corner
Biology Teaching Resources

Case Study: Cystic Fibrosis Mutations
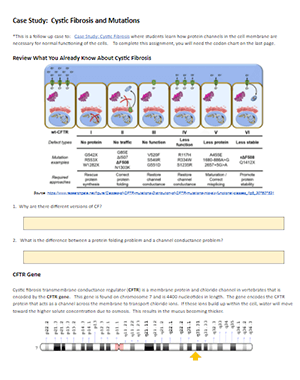
This case study is a follow-up to the Cystic Fibrosis Case Study where students explore how changes in transport proteins affects the movement of ions, resulting in a build-up of chloride ions and the symptoms of the disease.
Students were introduced to the idea that different mutations can cause differences in the transport proteins, but in the first version, the origin of these mutations was not discussed.
Eventually, students get to the chapter on DNA, RNA, and protein synthesis, so it’s a good time to circle back to the CF case and explore how mutations in DNA can affect the protein made by the ribosomes.
Students should already have some background in the central dogma, but a review may be in order to remind students how to transcribe DNA to RNA and then use a codon chart to determine the sequence of amino acids. This practice worksheet on using codon charts is something they may have done in freshman biology.
CFTR Mutations
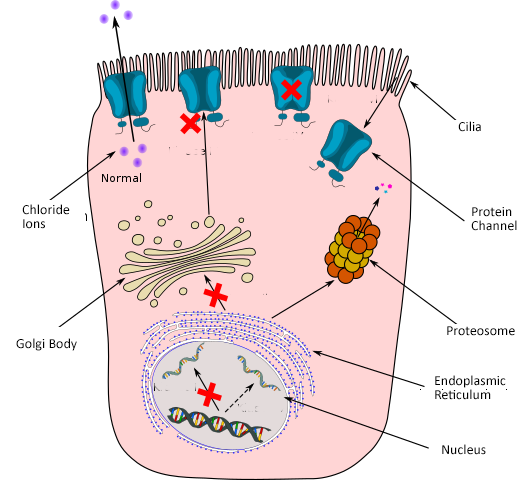
This case explore frameshift mutations, missense mutations, and nonsense mutations. Students are given a section of DNA to transcribe and compare it to mutant DNA. Students should see that changes in DNA can result in changes in the synthesized protein, though some changes are more profound than others.
The link below is a Google Doc designed for remote learning but will work for in-class lessons. An original in-class version is also available, where it doesn’t have the colored text boxes.
Shannan Muskopf
Cystic fibrosis occurs when the cystic fibrosis transmembrane conductance regulator (CFTR) protein is either not made correctly, or not made at all. By understanding how the protein is made, scientists have been able to develop treatments that target the protein and restore its function.
- The cystic fibrosis transmembrane conductance regulator (CFTR) protein helps to maintain the balance of salt and water on many surfaces in the body, such as the surface of the lung.
- The CFTR protein is a particular type of protein called an ion channel. In the lung, the CFTR ion channel moves chloride ions from inside the cell to outside the cell.
Researchers are still trying to learn more about the structure of the CFTR protein so that they can find new and better ways to help improve the function of the protein in people with CF.
The cystic fibrosis transmembrane conductance regulator (CFTR) protein helps to maintain the balance of salt and water on many surfaces in the body, such as the surface of the lung. When the protein is not working correctly, chloride — a component of salt — becomes trapped in cells. Without the proper movement of chloride, water cannot hydrate the cellular surface. This leads the mucus covering the cells to become thick and sticky, causing many of the symptoms associated with cystic fibrosis.
To understand how mutations in the CFTR gene cause the protein to become dysfunctional, it is important to understand how the protein is normally made, and how it helps to move water and chloride to the cell surface.
What Are Proteins?
Proteins are tiny machines that do specific jobs within a cell. The instructions for building each protein are encoded in DNA. Proteins are assembled from building blocks called amino acids. There are 20 different amino acids. All proteins are made up of chains of these amino acids connected together in different orders, like different words that are written using the same 26 letters of the alphabet. The DNA instructions tell the cell which amino acid to use at each position in the chain to make a specific protein.
The CFTR protein is made up of 1,480 amino acids. Once the CFTR protein chain is made, it is folded into a specific 3-D shape. The CFTR protein is shaped like a tube that goes through the membrane surrounding the cell, like a straw goes through the plastic top on a cup.

What Does the CFTR Protein Do?
The CFTR protein is a particular type of protein called an ion channel. An ion channel moves atoms or molecules that have an electrical charge from inside the cell to outside, or from outside the cell to inside. In the lung, the CFTR ion channel moves chloride ions from inside the cell to outside the cell. To get out of the cell, the chloride ions move through the center of the tube formed by the CFTR protein.
Once the chloride ions are outside the cell, they attract a layer of water. This water layer is important because it allows tiny hairs on the surface of the lung cells, called cilia, to sweep back and forth. This sweeping motion moves mucus up and out of the airways.
How Do Problems With the CFTR Protein Cause CF?
In people with CF, mutations in the CFTR gene can cause the following problems with the CFTR protein:
- It doesn't work well
- It isn't produced in sufficient quantities
- It is not produced at all
When any of these problems occur, the chloride ions are trapped inside the cell, and water is no longer attracted to the space outside the cell. When there is less water outside the cells, the mucus in the airways becomes dehydrated and thickens, causing it to flatten the cilia. The cilia can't sweep properly when thick, sticky mucus weighs them down.
Because the cilia can't move properly, mucus gets stuck in the airways, making it difficult to breathe. In addition, germs caught in the mucus are no longer expelled from the airway, allowing them to multiply and cause infections. Thick mucus in the lungs and frequent airway infections are some of the most common problems people with CF face.
Researchers Are Still Studying the Basic Structure
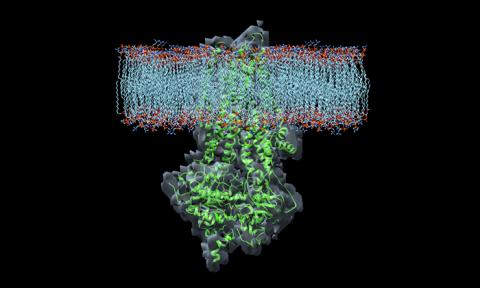
Because the 3-D shape of CFTR is so complex, it was not until early 2017 that the first high-resolution pictures were developed. These pictures have given researchers important clues about where drugs bind the protein, how they affect its function, and how to develop new CF therapies. In the future, pictures showing the protein in an “open” position, where salt can move through, will be even more helpful to researchers developing new CF therapies.
CF Genetics: The Basics Article | 6 min read
Types of CFTR Mutations Article | 9 min read
Restore CFTR: Exploring Treatments for Rare and Nonsense Mutations Article | 8 min read
Find Out More About Your Mutations Article | 3 min read
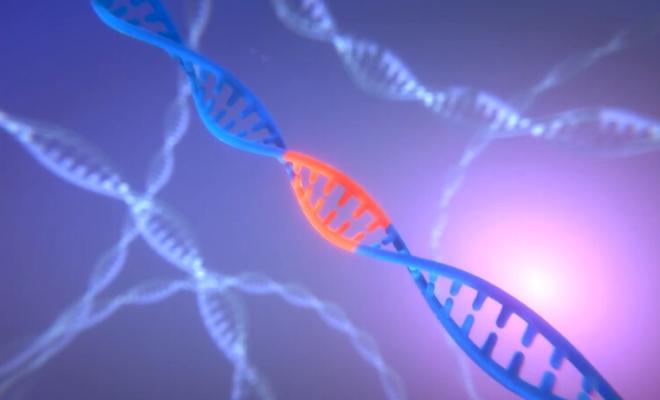
( 1-800-344-4823 ) Mon - Thu, 9 am - 7 pm ET Fri, 9 am - 3 pm ET
- Published: 16 December 2010
Protein biomarkers in cystic fibrosis research: where next?
- Sally H Pattison 1 &
- J Stuart Elborn 1
Genome Medicine volume 2 , Article number: 88 ( 2010 ) Cite this article
6241 Accesses
5 Citations
Metrics details
Cystic fibrosis is one of the most common life-limiting inherited disorders. Its clinical impact manifests chiefly in the lung, pancreas, gastrointestinal tract and sweat glands, with lung disease typically being most detrimental to health. The median age for survival has increased dramatically over the past decades, largely thanks to advances in understanding of the mechanisms and consequences of disease, leading to the development of better therapies and treatment regimes. The discovery of dysregulated protein biomarkers linked to cystic fibrosis has contributed considerably to this end. This article outlines clinical trials targeting known protein biomarkers, and the current and future contributions of proteomic techniques to cystic fibrosis research. The treatments described range from those designed to provide functional copies of the mutant protein responsible for cystic fibrosis, to others addressing the associated symptoms of chronic inflammation. Preclinical research has employed proteomics to help elucidate pathways and processes implicated in disease that might present opportunities for therapy or prognosis. Global analyses of cystic fibrosis have detected the differential expression of proteins involved in inflammation, proteolytic activity and oxidative stress, which are recognized symptoms of the cystic fibrosis phenotype. The dysregulation of other processes, such as the complement and mitochondrial systems, has also been implicated. A number of studies have focused specifically on proteins that interact with the cystic fibrosis protein, with the goal of restoring its normal proteostasis. Consequently, proteins involved in synthesis, folding, degradation, translocation and localization of the protein have been identified as potential therapeutic targets. Cystic fibrosis patients are prone to lung infections that are thought to contribute to chronic inflammation, and thus proteomic studies have also searched for microbiological biomarkers to use in early infection diagnosis or as indicators of virulence. The review concludes by proposing a future role for proteomics in the high-throughput validation of protein biomarkers under consideration as outcome measures for use in clinical trials and routine disease monitoring.
Cystic fibrosis pathophysiology
Cystic fibrosis (CF) is a recessive disorder caused by mutations to the gene encoding the cystic fibrosis transmembrane conductance regulator ( CFTR ), a chloride channel responsible for directing the movement of salt and water in and out of cells. To date, over 1,700 mutations of this gene [ 1 ], categorized into six classes according to their functional effects [ 2 ], have been linked to CF. F508del is the most common mutation and accounts for 70% of CF in most Caucasian populations [ 3 ].
The reduction of CFTR activity perturbs normal water and salt balance, resulting in the production of unusually thick, sticky mucus at tissue surfaces. This reduction in surface fluid in the lung promotes persistent and recurrent infections by impeding the mucociliary clearance of invading microbes [ 4 ], and in the digestive tract by hindering the movement of feces, which may cause bowel obstruction. Mucus plugging in the glands may block ducts, leading to tissue scarring and loss of function. In particular, ductal plugging of the pancreas prevents the production of sufficient digestive enzymes for effective nutrition.
Protein targets in current CF clinical trials
Following the cloning of the CF gene in 1989 [ 5 ] and the subsequent introduction of DNA-based disease diagnosis, the focus of protein target discovery shifted towards the identification of candidate drug targets and indicators of disease severity and/or response to treatment. Restoration of normal CFTR function is the chief therapeutic goal as this will address the underlying cause of CF disease rather than just treat the symptoms. Gene therapy trials are underway aiming to deliver functional CFTR genes to the epithelial cells of the CF airway. In phase 1, treatment with aerosolized compacted DNA nanoparticles containing the CFTR gene (Copernicus Therapeutics, Cleveland, Ohio, USA) induced nasal chloride current changes in CF patients, suggesting increased CFTR functionality, but gene expression was not detected [ 6 ]. The UK Cystic Fibrosis Gene Therapy Consortium [ 7 ] is currently performing a phase 1/2 safety study using pGM169/GL67A [ 8 ] and will proceed to a multidose study in July 2011. This system utilizes liposomes to promote the aerosolized delivery of a DNA plasmid containing the CFTR gene.
Two drugs from Vertex Pharmaceuticals (Abingdon, UK), VX-770 and VX-809, aiming to promote the activity of mutant CFTR by increasing channel opening and trafficking to the membrane, respectively, are currently in clinical trials [ 6 ]. In phase 2, VX-770 improved measures of CFTR function such as nasal potential difference and sweat chloride concentration [ 6 ]. Ataluren (formerly PTC124; PTC Therapeutics, South Plainfield, New Jersey, USA), which is designed to increase synthesis of full-length functional CFTR , improved CFTR function for some patients in phase 2 trials [ 9 ] and is currently in phase 3 [ 6 ]. Sildenafil, which corrects F508del- CFTR trafficking and increased chloride transport in F508del- CFTR mice [ 10 ], is the subject of phase 1/2 clinical trials [ 8 ].
Lung disease, resulting from chronic infection and inflammation, is the most common cause of death in the CF population and thus its treatment is a key goal of CF therapy. In the CF lung, activation of the nuclear factor (NF)-κB signaling pathway leads to enhanced production of pro-inflammatory mediators, including interleukin (IL)-8. IL-8 is a potent neutrophil chemoattractant resulting in neutrophil recruitment and accompanying tissue damage through the release of neutrophil proteases and reactive oxygen species. Drugs are being developed to treat various proteins involved in this inflammatory cycle. Digitoxin has been shown to suppress hypersecretion of pro-inflammatory IL-8 by CF lung epithelial cells in vitro [ 11 ] and its effect on sputum IL-8 and neutrophil counts is currently being assessed in a phase 2 clinical trial (ClinicalTrials.gov Identifier: NCT00782288 [ 8 ]). GSK SB 656933 (GlaxoSmithKline, Uxbridge, UK) is an antagonist of the neutrophil IL-8 receptor CXCR2, which mediates neutrophil migration. It has demonstrated safety in a phase 1 trial [ 6 ] and is now being evaluated in a phase 2 study (ClinicalTrials.gov Identifier: NCT00903201 [ 8 ]) for pharmacodynamics and efficacy, including the reduction of sputum neutrophil elastase and neutrophil counts. Pioglitazone, already approved for treatment of other clinical conditions, is being assessed for safety and anti-inflammatory action in phase 1 clinical trials against CF lung disease [ 6 ]. Its target, peroxisome proliferator-activated receptor γ, which is reduced in CF [ 12 ], exerts an anti-inflammatory effect by negatively regulating NF-κB activation [ 13 ]. The sputum protease matrix metalloproteinase-9 has also been linked to poor lung function and airway inflammation in CF children [ 14 ] and its activity is being targeted by the antibiotic doxycycline in a current trial (ClinicalTrials.gov Identifier: NCT01112059 [ 8 ]).
Various proteins and protein degradation products have been explored as candidate biomarkers of clinical outcome, such as neutrophil elastase and IL-8 [ 15 ], degradation of lung surfactant protein SP-A [ 16 ], urinary desmosine [ 17 ] and proline-glycine-proline [ 18 ]. However, as yet, none of these markers has been proven sufficiently robust for routine adoption in clinical trials [ 19 ].
Proteomic contributions to CF research
Preclinical medical research is increasingly adopting a systems rather than a reductionist approach to understanding and treating disease, with clinical proteomics contributing to the characterization and measurement of pathophysiological stages. Proteomic-based CF research has employed techniques such as two-dimensional gel electrophoresis (2-DE), liquid chromatography, mass spectrometry (MS) and antibody/protein microarrays to analyze secretions, cells and whole tissues from in vitro or in vivo disease models, human subjects and infecting micro-organisms. Laser capture microdissection, cell fractionation and co-immunoprecipitation have been used to limit analyses to the sub-proteomes of interest.
Global comparative analyses of CF versus non-CF samples have been used to identify differentially expressed proteins in human bronchoalveolar lavage fluid (BALF) [ 20 , 21 ], sputum [ 22 ], bronchial biopsy tissue [ 23 ], serum [ 24 ] and cultured epithelial cells [ 25 , 26 ], and in mouse lung and colonic tissue [ 27 – 29 ]. Many of the proteins highlighted by global analyses can be related functionally to biological processes and pathways known to contribute to CF disease pathogenesis, including chronic inflammation, proteolytic activity and oxidative stress response proteins.
Chronic neutrophil-mediated inflammation typifies the CF lung, and comparative proteomic studies have provided data to support and improve our understanding of the mechanisms involved. Srivastava et al . [ 24 ] have detected in CF serum differential levels of proteins belonging to the NF-κB pathway, which is known to enhance production of inflammatory mediators, while Sloane et al . [ 30 ] have found that sputum from adults with CF is characterized by inflammation-related proteins, including increased production of IL-8. Neutrophil proteins, including α-defensins and S100 proteins, have been shown to be differentially expressed in CF BALF [ 31 ] and sputum [ 22 ]. Also, lower levels of anti-inflammatory proteins Clara cell secretory protein [ 22 ] and annexin A1 [ 29 ] have been observed in CF nasal epithelial cells and sputum, respectively. Additionally, the absence of annexin A1 has been associated with upregulation of the proinflammatory cytosolic phospholipase A2 in the colonic crypts of CF mice [ 29 ].
Chronic inflammation of the CF lung is thought to induce the overexpression of proteases, thus perturbing the protease/anti-protease balance and resulting in tissue damage and disease. Through the application of shotgun proteomic methods, Gharib et al . [ 20 ] detected increased levels of 22 proteases and peptidases in human CF BALF, including neutrophil elastase, cathepsin G and proteinase 3. They also identified increased expression of human monocyte/neutrophil elastase inhibitor [ 20 ], which when applied as an aerosolized treatment to rats has been shown to reduce inflammation [ 32 ]. Extensive proteolytic degradation, including truncation of the anti-protease α 1 -antitrypsin and degradation of IgG, has been observed in CF sputum [ 30 ].
High levels of toxic reactive oxygen species and oxidative stress are characteristic of the CF lung. Reduced glutathione, which acts as an antioxidant and in detoxification, has been observed at a lower level in CF lung lavage fluid [ 33 ]. In support of this finding, Roxo-Rosa et al . [ 26 ] have detected, via 2-DE comparative proteomics of CF and non-CF mouse nasal epithelial cells, reductions in the levels of glutathione-related proteins: glutathione S -transferase, which catalyses the glutathione-mediated detoxification of oxidative stress products; peroxiredoxin 6, a glutathione-dependent peroxidase involved in defense against oxidative stress; and Hsp27, a heat shock protein that can increase intracellular levels of glutathione and acts as a chaperone for detoxification. Other proteomic studies have identified differential expression of myeloperoxidase, superoxide dismutase, catalase and glutathione reductase in CF BALF [ 20 ], and increased levels of myeloperoxidase in CF sputum [ 30 ]. Together these data help elucidate mechanisms that are likely to contribute to oxidative stress in the CF lung.
Other biological processes and proteins where functional links to CF disease are less well established or absent have also been implicated by global comparative proteomics. Differential expression of mitochondrial proteins has been reported in human CF nasal epithelial cells [ 26 ] and bronchial tissue [ 23 ], implicating a CF-associated reduction in mitochondrial metabolism, and the recent mapping of the CF BALF proteome [ 20 ] has implicated dysregulation of the complement system as a novel CF phenotype that may impact lung disease pathogenesis by impairing response to chronic infections. Investigation of the response of murine CF airway epithelial cells to injury detected reductions in enzymes involved in prostaglandin and retinoic acid metabolism; this implicates these pathways in the CF abnormal injury response, although no functional role has been determined.
More focused comparative proteomic studies have concentrated on specific protein subgroups, such as those involved in pathways of interest or executing certain roles. An investigation by Chen et al . [ 34 ] of the mechanisms triggering the overproduction of cytokines IL-6 and IL-8, associated with excessive CF lung inflammation, identified a regulatory pathway that is significantly reduced in CF. Moreover, they demonstrated that correction of the pathway reduced IL-6 and IL-8 production [ 34 ]. Individual protein families have also been studied, such as lung surfactant proteins and mucins, both of which are involved in pathogen clearance from the airways. The structural modification of lung surfactant proteins SP-A and SP-D [ 35 ] and degradation of mucins MUC5B and MUC5AC [ 36 ] have been detected in CF BALF and sputum, respectively, and are thought to be relevant to lung disease. Additionally, mucin glycosylation has been highlighted as a possible predictor of lung condition [ 36 ].
Particular attention has been directed towards identifying proteins that interact with CFTR with the goal of understanding and restoring normal CFTR proteostasis through the correction of CFTR synthesis, folding, aggregation, degradation, trafficking and stable localization. F508del, the most common mutation of the CFTR gene, gives rise to incorrectly folded CFTR that is translocated from the endoplasmic reticulum to the cytosol for proteosomal degradation. An investigation by Goldstein et al . [ 37 ] of proteins that co-precipitate with F508del- CFTR has identified interaction with valosin-containing protein (VCP)/p9, a component of the translocation machinery, as being associated with inefficient processing of the mutant CFTR . Gomes-Alves et al . [ 38 ] used 2-DE to compare protein profiles of cell lines expressing wild-type or F508del- CFTR at 37°C and 26°C, and have identified mechanisms, including the induction of the unfolded protein response and downregulation of degradative proteins, which may contribute to the cold-shock-induced rescue of F508del- CFTR . By comparing the CFTR interactomes of bronchial epithelial cells expressing chemically and genetically repaired F508del- CFTR , Singh et al . [ 39 ] identified a set of Hsp70 family proteins as implicated in rescue of the mutant protein. Additionally, Wang et al . [ 40 ] showed the importance of Hsp60 co-chaperones in CFTR folding and demonstrated rescue of F508del- CFTR by partial small interfering RNA silencing of the Hsp60 co-chaperone Aha1. Understanding the mechanisms that can contribute to F508del- CFTR rescue may suggest potential therapeutic targets. Also, study of the CFTR interactome has led to elucidation of the molecular defect of S13F- CFTR [ 41 ] as relating to defective interaction with filamins, which anchor plasma membrane CFTR to the actin cytoskeleton [ 41 ].
Repeated or chronic microbial infection is thought to be a major contributor to the excessive inflammation that precipitates CF lung damage, and a variety of proteomic approaches have been exploited to discover bacterial antigenic biomarkers that could provide potential candidates for infection diagnosis, prognosis indicators or vaccine development. Pedersen et al . [ 42 ] used a novel enrichment technique employing CF patient antibodies as capture ligands prior to proteomic analysis to enhance the identification of Pseudomonas aeruginosa antigens. The antigens detected by this method included stress, immunosuppressive and alginate synthetase pathway proteins. Using proteomic analysis of non-enriched serum samples from CF patients with different stages of infection, Rao et al . [ 43 ] identified outer membrane protein OprL as associated with initial P. aeruginosa infection and thus proposed serum reactivity to OprL as an early diagnostic. Montor et al . [ 44 ] generated protein microarrays displaying all predicted outer membrane and exported proteins expressed by P. aeruginosa reference strain PAO1 and used these to interrogate serum samples from CF patients infected with P. aeruginosa . They identified 48 antigenic proteins, 12 of which were common to approximately 50% of the samples. Alternatively, whole cell MS has been proposed for the rapid identification of commonly misidentified bacterial species [ 45 ].
Proteomics has helped elucidate factors pertinent to virulence, adaptation and in vivo survival of the pathogen P. aeruginosa [ 46 – 49 ], which is particularly indicative of a poor prognosis [ 50 ]. These may present candidate drug targets for treatment of CF infections. The quorum sensing intercellular communication systems have received particular attention as they largely coordinate bacterial virulence [ 51 , 52 ].
The future potential of proteomics
The constant advance of proteomic strategies, instrumentation and data analysis provides an ever-increasing set of tools available for expanding CF research. Two strands for future studies are envisaged: the continuing investigation of disease pathology aiming to discover prognostic, diagnostic and therapeutic biomarkers; and the translation of existing knowledge into clinical applications of benefit to the CF population. The recent drift from traditional 2-DE to gel-free methods for protein and peptide separation is still under-represented in CF biomarker discovery, although their potential for extending shotgun (that is, global) proteome coverage has already been demonstrated for BALF. By complementing traditional gel separation of proteins with the two-dimensional liquid chromatography separation of tryptic digests, Guo et al . [ 53 ] were able to improve the number of proteins detected in mouse BALF from 212 to 297, although they noted that their methods did not permit quantitative analyses. More recently, Gharib et al . [ 20 ] used quantitative shotgun proteomics to compare human CF and non-CF BALF from 12 subjects, and were able to distinguish the differential expression of hundreds of proteins, including those involved in pathways implicated in CF pathophysiology. Wider adoption of these approaches will enable protein detection over a larger dynamic range, thus including in the pool of potential biomarkers proteins of lower abundance that could not be detected using traditional proteomic techniques. Future biomarker discovery will also greatly benefit from the recent generation of pigs with mutated CFTR genes [ 54 , 55 ], which provides a model that more closely resembles human disease than current mouse models, and thus enables further investigation of biomarkers relevant to long-term disease progression and treatment efficacy.
The major shortfall in current proteomics research is the gap between the discovery of biomarkers and their clinical application. One hindrance has been the lack of tools for high-throughput validation. Advances in MS selected reaction monitoring have enabled the concurrent measurement of multiple researcher-designated proteins in a sample and may in future bypass the need for the development of a separate antibody-based assay for each individual proteins to be quantified [ 56 ]. This increases the feasibility of assaying large sample numbers with sufficiently high specificity and sensitivity to enable the simultaneous statistical validation of multiple candidate biomarkers [ 57 ]. The validation of a panel of CF-specific protein biomarkers could precipitate the production of novel biomarker arrays or tests for individual proteins. Such tools would permit the quantification of new outcome measures for assessing disease progression and/or response to treatment in clinical trials, and may be applicable to future routine clinical practice [ 58 ].
Conclusions
Proteins and their interactions ultimately steer CF disease, making their study invaluable for improving our understanding of pathophysiology and potential treatment opportunities. Considerable knowledge has been gained so far by the application of proteomics to CF research and rapid advancements in this field are expected to augment its future contribution towards improving the prognosis of CF patients.
Abbreviations
bronchoalveolar lavage fluid
cystic fibrosis
cystic fibrosis transmembrane conductance regulator
two-dimensional electrophoresis
interleukin
mass spectrometry
nuclear factor.
Cystic Fibrosis Mutation Database. --- Either ISSN or Journal title must be supplied.. [ http://www.genet.sickkids.on.ca/cftr ]
Ratjen FA: Cystic fibrosis: pathogenesis and future treatment strategies. Respir Care. 2009, 54: 595-605. 10.4187/aarc0427.
Article PubMed Google Scholar
Cystic Fibrosis Genetic Analysis Consortium: Worldwide survey of the delta F508 mutation - report from the cystic fibrosis genetic analysis consortium. Am J Hum Genet. 1990, 47: 354-359.
Google Scholar
Boucher R: Evidence for airway surface dehydration as the initiating event in CF airway disease. J Intern Med. 2007, 261: 5-16. 10.1111/j.1365-2796.2006.01744.x.
Article PubMed CAS Google Scholar
Riordan J, Rommens J, Kerem B, Alon N, Rozmahel R, Grzelczak Z, Zielenski J, Lok S, Plavsic N, Chou J: Identification of the cystic fibrosis gene: cloning and characterization of complementary DNA. Science. 1989, 245: 1066-1073. 10.1126/science.2475911.
Cystic Fibrosis Foundation. --- Either ISSN or Journal title must be supplied.. [ http://www.cff.org ]
The UK Cystic Fibrosis Gene Therapy Consortium. --- Either ISSN or Journal title must be supplied.. [ http://www.cfgenetherapy.org.uk ]
ClinicalTrials.gov. --- Either ISSN or Journal title must be supplied.. [ http://www.clinicaltrials.gov ]
Kerem E, Hirawat S, Armoni S, Yaakov Y, Shoseyov D, Cohen M, Nissim-Rafinia M, Blau H, Rivlin J, Aviram M, Elfring GL, Northcutt VJ, Miller LL, Kerem B, Wilschanski M: Effectiveness of PTC124 treatment of cystic fibrosis caused by nonsense mutations: a prospective phase II trial. Lancet. 2008, 372: 719-727. 10.1016/S0140-6736(08)61168-X.
Lubamba B, Lecourt H, Lebacq J, Lebecque P, De Jonge H, Wallemacq P, Leal T: Preclinical evidence that sildenafil and vardenafil activate chloride transport in cystic fibrosis. Am J Respir Crit Care Med. 2008, 177: 506-515. 10.1164/rccm.200703-344OC.
Srivastava M, Eidelman O, Zhang J, Paweletz C, Caohuy H, Yang Q, Jacobson KA, Heldman E, Huang W, Jozwik C, Pollard BS, Pollard HB: Digitoxin mimics gene therapy with CFTR and suppresses hypersecretion of IL-8 from cystic fibrosis lung epithelial cells. Proc Natl Acad Sci USA. 101: 7693-7698. 10.1073/pnas.0402030101.
Ollero M, Junaidi O, Zaman MM, Tzameli I, Ferrando AA, Andersson C, Blanco PG, Bialecki E, Freedman SD: Decreased expression of peroxisome proliferator activated receptor γ in CFTR -/- mice. J Cell Physiol. 2004, 200: 235-244. 10.1002/jcp.20020.
Perez A, van Heeckeren AM, Nichols D, Gupta S, Eastman JF, Davis PB: Peroxisome proliferator-activated receptor-γ in cystic fibrosis lung epithelium. Am J Physiol Lung Cell Mol Physiol. 2008, 295: L303-L313. 10.1152/ajplung.90276.2008.
Article PubMed CAS PubMed Central Google Scholar
Sagel S, Kapsner R, Osberg L: Induced sputum matrix metalloproteinase-9 correlates with lung function and airway inflammation in children with cystic fibrosis. Pediatr Pulmonol. 2005, 39: 224-232. 10.1002/ppul.20165.
Mayer-Hamblett N, Aitken ML, Accurso FJ, Kronmal RA, Konstan MW, Burns JL, Sagel SD, Ramsey BW: Association between pulmonary function and sputum biomarkers in cystic fibrosis. Am J Respir Crit Care Med. 2007, 175: 822-828. 10.1164/rccm.200609-1354OC.
Article PubMed PubMed Central Google Scholar
Griese M, von Bredow C, Birrer P: Reduced proteolysis of surfactant protein A and changes of the bronchoalveolar lavage fluid proteome by inhaled alpha 1-protease inhibitor in cystic fibrosis. Electrophoresis. 2001, 22: 165-171. 10.1002/1522-2683(200101)22:1<165::AID-ELPS165>3.0.CO;2-H.
Downey D, Martin S, Dempster M, Moore J, Keogan M, Starcher B, Edgar J, Bilton D, Elborn J: The relationship of clinical and inflammatory markers to outcome in stable patients with cystic fibrosis. Pediatr Pulmonol. 2007, 42: 216-220. 10.1002/ppul.20553.
Rowe SM, Jackson PL, Liu G, Hardison M, Livraghi A, Solomon GM, McQuaid DB, Noerager BD, Gaggar A, Clancy JP, O'Neal W, Sorscher EJ, Abraham E, Blalock JE: Potential role of high-mobility group box 1 in cystic fibrosis airway disease. Am J Respir Crit Care Med. 2008, 178: 822-831. 10.1164/rccm.200712-1894OC.
Sagel SD, Chmiel JF, Konstan MW: Sputum biomarkers of inflammation in cystic fibrosis lung disease. Proc Am Thorac Soc. 2007, 4: 406-417. 10.1513/pats.200703-044BR.
Gharib S, Vaisar T, Aitken M, Park D, Heinecke J, Fu X: Mapping the lung proteome in cystic fibrosis. J Proteome Res. 2009, 8: 3020-3029. 10.1021/pr900093j.
MacGregor G, Gray RD, Hilliard TN, Imrie M, Boyd AC, Alton EWFW, Bush A, Davies JC, Innes JA, Porteous DJ, Greening AP: Biomarkers for cystic fibrosis lung disease: application of SELDI-TOF mass spectrometry to BAL fluid. J Cyst Fibros. 2008, 7: 352-358. 10.1016/j.jcf.2007.12.005.
Gray RD, MacGregor G, Noble D, Imrie M, Dewar M, Boyd AC, Innes JA, Porteous DJ, Greening AP: Sputum proteomics in inflammatory and suppurative respiratory diseases. Am J Respir Crit Care Med. 2008, 178: 444-452. 10.1164/rccm.200703-409OC.
Frischer T, Myung J, Maurer G, Eichler I, Szepfalusi Z, Lubec G: Possible dysregulation of chaperon and metabolic proteins in cystic fibrosis bronchial tissue. Proteomics. 2006, 6: 3381-3388. 10.1002/pmic.200500487.
Srivastava M, Eidelman O, Jozwik C, Paweletz C, Huang W, Zeitlin PL, Pollard HB: Serum proteomic signature for cystic fibrosis using an antibody microarray platform. Mol Genet Metab. 2006, 87: 303-310. 10.1016/j.ymgme.2005.10.021.
Pollard HB, Eidelman O, Jozwik C, Huang W, Srivastava M, Ji XD, McGowan B, Norris CF, Todo T, Darling T, Mogayzel PJ, Zeitlin PL, Wright J, Guggino WB, Metcalf E, Driscoll WJ, Mueller G, Paweletz C, Jacobowitz DM: De novo biosynthetic profiling of high abundance proteins in cystic fibrosis lung epithelial cells. Mol Cell Proteomics. 2006, 5: 1628-1637. 10.1074/mcp.M600091-MCP200.
Roxo-Rosa M, da Costa G, Luider T, Scholte B, Coelho A, Amaral M, Penque D: Proteomic analysis of nasal cells from cystic fibrosis patients and non-cystic fibrosis control individuals: search for novel biomarkers of cystic fibrosis lung disease. Proteomics. 2006, 6: 2314-2325. 10.1002/pmic.200500273.
Carvalho-Oliveira I, Charro N, Aarbiou J, Buijs-Offerman R, Wilke M, Schettgen T, Kraus T, Titulaer M, Burgers P, Luider T, Penque D, Scholte B: Proteomic analysis of naphthalene-induced airway epithelial injury and repair in a cystic fibrosis mouse model. J Proteome Res. 2009, 8: 3606-3616. 10.1021/pr900021m.
Brouillard F, Bensalem N, Hinzpeter A, Tondelier D, Trudel S, Gruber AD, Ollero M, Edelman A: Blue native/SDS-PAGE analysis reveals reduced expression of the mClCA3 protein in cystic fibrosis knock-out mice. Mol Cell Proteomics. 2005, 4: 1762-1775. 10.1074/mcp.M500098-MCP200.
Bensalem N, Ventura AP, Vallée B, Lipecka J, Tondelier D, Davezac N, Santos AD, Perretti M, Fajac A, Sermet-Gaudelus I, Renouil M, Lesure J, Halgand F, Laprévote O, Edelman A: Down-regulation of the anti-inflammatory protein annexin A1 in cystic fibrosis knock-out mice and patients. Mol Cell Proteomics. 2005, 4: 1591-1601. 10.1074/mcp.M500019-MCP200.
Sloane AJ, Lindner RA, Prasad SS, Sebastian LT, Pedersen SK, Robinson M, Bye PT, Nielson DW, Harry JL: Proteomic analysis of sputum from adults and children with cystic fibrosis and from control subjects. Am J Respir Crit Care Med. 2005, 172: 1416-1426. 10.1164/rccm.200409-1215OC.
McMorran BJ, Ouvry Patat SA, Carlin JB, Grimwood K, Jones A, Armstrong DS, Galati JC, Cooper PJ, Byrnes CA, Francis PW, Robertson CF, Hume DA, Borchers CH, Wainwright CE, Wainwright BJ: Novel neutrophil-derived proteins in bronchoalveolar lavage fluid indicate an exaggerated inflammatory response in pediatric cystic fibrosis patients. Clin Chem. 2007, 53: 1782-1791. 10.1373/clinchem.2007.087650.
Woods DE, Cantin A, Cooley J, Kenney DM, Remold-O'Donnell E: Aerosol treatment with MNEI suppresses bacterial proliferation in a model of chronic Pseudomonas aeruginosa lung infection. Pediatr Pulmonol. 2005, 39: 141-149. 10.1002/ppul.20167.
Roum JH, Buhl R, McElvaney NG, Borok Z, Crystal RG: Systemic deficiency of glutathione in cystic fibrosis. J Appl Physiol. 1993, 75: 2419-2424.
PubMed CAS Google Scholar
Chen J, Kinter M, Shank S, Cotton C, Kelley T, Ziady A: Dysfunction of Nrf-2 in CF epithelia leads to excess intracellular H 2 O 2 and inflammatory cytokine production. PLoS One. 2008, 3: e3367-10.1371/journal.pone.0003367.
Bai Y, Galetskiy D, Damoc E, Paschen C, Liu Z, Griese M, Liu S, Przybylsk M: High resolution mass spectrometric alveolar proteomics: identification of surfactant protein SP-A and SP-D modifications in proteinosis and cystic fibrosis patients. Proteomics. 2004, 4: 2300-2309. 10.1002/pmic.200400855.
Schulz BL, Sloane AJ, Robinson LJ, Prasad SS, Lindner RA, Robinson M, Bye PT, Nielson DW, Harry JL, Packer NH, Karlsson NG: Glycosylation of sputum mucins is altered in cystic fibrosis patients. Glycobiology. 2007, 17: 698-712. 10.1093/glycob/cwm036.
Goldstein RF, Niraj A, Sanderson TP, Wilson LS, Rab A, Kim H, Bebok Z, Collawn JF: VCP/p97 AAA-ATPase does not interact with the endogenous wild-type cystic fibrosis transmembrane conductance regulator. Am J Respir Cell Mol Biol. 2007, 36: 706-714. 10.1165/rcmb.2006-0365OC.
Gomes-Alves P, Neves S, Coelho A, Penque D: Low temperature restoring effect on F508del-CFTR misprocessing: a proteomic approach. J Proteomics. 2009, 73: 218-230. 10.1016/j.jprot.2009.09.001.
Singh OV, Pollard HB, Zeitlin PL: Chemical rescue of ΔF508-CFTR mimics genetic repair in cystic fibrosis bronchial epithelial cells. Mol Cell Proteomics. 2008, 7: 1099-1110. 10.1074/mcp.M700303-MCP200.
Wang X, Venable J, LaPointe P, Hutt D, Koulov A, Coppinger J, Gurkan C, Kellner W, Matteson J, Plutner H, Riordan J, Kelly J, Yates J, Balch W: Hsp90 cochaperone Aha1 downregulation rescues misfolding of CFTR in cystic fibrosis. Cell. 2006, 127: 803-815. 10.1016/j.cell.2006.09.043.
Thelin W, Che Y, Gentzsch M, Kreda S, Sallee J, Scarlett C, Borchers C, Jacobson K, Stutts M, Milgram S: Direct interaction with filamins modulates the stability and plasma membrane expression of CFTR. J Clin Invest. 2007, 117: 364-374. 10.1172/JCI30376.
Pedersen SK, Sloane AJ, Prasad SS, Sebastian LT, Lindner RA, Hsu M, Robinson M, Bye PT, Weinberger RP, Harry JL: An immunoproteomic approach for identification of clinical biomarkers for monitoring disease. Mol Cell Proteomics. 2005, 4: 1052-1060. 10.1074/mcp.M400175-MCP200.
Rao AR, Laxova A, Farrell PM, Barbieri JT: Proteomic identification of OprL as a seromarker for initial diagnosis of Pseudomonas aeruginosa infection of patients with cystic fibrosis. J Clin Microbiol. 2009, 47: 2483-2488. 10.1128/JCM.02182-08.
Montor WR, Huang J, Hu Y, Hainsworth E, Lynch S, Kronish J, Ordonez CL, Logvinenko T, Lory S, LaBaer J: Genome-wide study of Pseudomonas aeruginosa outer membrane protein immunogenicity using self-assembling protein microarrays. Infect Immun. 2009, 77: 4877-4886. 10.1128/IAI.00698-09.
Vanlaere E, Sergeant K, Dawyndt P, Kallow W, Erhard M, Sutton H, Dare D, Devreese B, Samyn B, Vandamme P: Matrix-assisted laser desorption ionisation-time-of-flight mass spectrometry of intact cells allows rapid identification of Burkholderia cepacia complex. J Microbiol Methods. 2008, 75: 279-286. 10.1016/j.mimet.2008.06.016.
Sriramulu D, Nimtz M, Romling U: Proteome analysis reveals adaptation of Pseudomonas aeruginosa to the cystic fibrosis lung environment. Proteomics. 2005, 5: 3712-3721. 10.1002/pmic.200401227.
Malhotra S, Silo-Suh LA, Mathee K, Ohman DE: Proteome analysis of the effect of mucoid conversion on global protein expression in Pseudomonas aeruginosa strain PAO1 shows induction of the disulfide bond isomerase, DsbA. J Bacteriol. 2000, 182: 6999-7006. 10.1128/JB.182.24.6999-7006.2000.
Platt MD, Schurr MJ, Sauer K, Vazquez G, Kukavica-Ibrulj I, Potvin E, Levesque RC, Fedynak A, Brinkman FSL, Schurr J, Hwang S, Lau GW, Limbach PA, Rowe JJ, Lieberman MA, Barraud N, Webb J, Kjelleberg S, Hunt DF, Hassett DJ: Proteomic, microarray, and signature-tagged mutagenesis analyses of anaerobic Pseudomonas aeruginosa at pH 6.5, likely representing chronic, late-stage cystic fibrosis airway conditions. J Bacteriol. 2008, 190: 2739-2758. 10.1128/JB.01683-07.
Guina T, Purvine SO, Yi EC, Eng J, Goodlett Dr, Aebersold R: Quantitative proteomic analysis indicates increased synthesis of a quinolone by Pseudomonas aeruginosa isolates from cystic fibrosis airways. Proc Natl Acad Sci USA. 2003, 100: 2771-2776. 10.1073/pnas.0435846100.
Courtney J, Bradley J, McCaughan J, O'Connor T, Shortt C, Bredin C, Bradbury I, Elborn J: Predictors of mortality in adults with cystic fibrosis. Pediatr Pulmonol. 2007, 42: 525-532. 10.1002/ppul.20619.
Arevalo-Ferro C, Hentzer M, Reil G, Görg A, Kjelleberg S, Givskov M, Riedel K, Eberl L: Identification of quorum-sensing regulated proteins in the opportunistic pathogen Pseudomonas aeruginosa by proteomics. Environ Microbiol. 2003, 5: 1350-1369. 10.1046/j.1462-2920.2003.00532.x.
Riedel K, Arevalo-Ferro C, Reil G, Görg A, Lottspeich F, Eberl L: Analysis of the quorum-sensing regulon of the opportunistic pathogen Burkholderia cepacia H111 by proteomics. Electrophoresis. 2003, 24: 740-750. 10.1002/elps.200390089.
Guo Y, Ma S, Grigoryev D, Van Eyk J, Garcia J: 1-DE MS and 2-D LC-MS analysis of the mouse bronchoalveolar lavage proteome. Proteomics. 2005, 5: 4608-4624. 10.1002/pmic.200500052.
Welsh M, Rogers C, Stoltz D, Meyerholz D, Prather R: Development of a porcine model of cystic fibrosis. Trans Am Clin Climatol Assoc. 2009, 120: 149-162.
PubMed PubMed Central Google Scholar
Stoltz DA, Meyerholz DK, Pezzulo AA, Ramachandran S, Rogan MP, Davis GJ, Hanfland RA, Wohlford-Lenane C, Dohrn CL, Bartlett JA, Nelson GA, Chang EH, Taft PJ, Ludwig PS, Estin M, Hornick EE, Launspach JL, Samuel M, Rokhlina T, Karp PH, Ostedgaard LS, Uc A, Starner TD, Horswill AR, Brogden KA, Prather RS, Richter SS, Shilyansky J, McCray PB, Zabner J, Welsh MJ: Cystic fibrosis pigs develop lung disease and exhibit defective bacterial eradication at birth. Sci Transl Med. 2010, 2: 29ra31-29ra31.
Kitteringham NR, Jenkins RE, Lane CS, Elliott VL, Park BK: Multiple reaction monitoring for quantitative biomarker analysis in proteomics and metabolomics. J Chromatogr B Analyt Technol Biomed Life Sci. 2009, 877: 1229-1239. 10.1016/j.jchromb.2008.11.013.
Makawita S, Diamandis EP: The bottleneck in the cancer biomarker pipeline and protein quantification through mass spectrometry-based approaches: current strategies for candidate verification. Clin Chem. 2010, 56: 212-222. 10.1373/clinchem.2009.127019.
Ramsey BW: Outcome measures for development of new therapies in cystic fibrosis: are we making progress and what are the next steps?. Proc Am Thorac Soc. 2007, 4: 367-369. 10.1513/pats.200703-038BR.
Download references
Author information
Authors and affiliations.
Centre for Infection and Immunity, Queen's University Belfast, Belfast, BT9 7BL, Northern Ireland, UK
Sally H Pattison & J Stuart Elborn
You can also search for this author in PubMed Google Scholar
Corresponding author
Correspondence to J Stuart Elborn .
Additional information
Competing interests.
The authors declare that they have no competing interests.
Authors' contributions
SHP and JSE drafted and approved the manuscript.
Rights and permissions
Reprints and permissions
About this article
Cite this article.
Pattison, S.H., Elborn, J.S. Protein biomarkers in cystic fibrosis research: where next?. Genome Med 2 , 88 (2010). https://doi.org/10.1186/gm209
Download citation
Published : 16 December 2010
DOI : https://doi.org/10.1186/gm209
Share this article
Anyone you share the following link with will be able to read this content:
Sorry, a shareable link is not currently available for this article.
Provided by the Springer Nature SharedIt content-sharing initiative
- Cystic Fibrosis
- Cystic Fibrosis Transmembrane Conductance Regulator
- Cystic Fibrosis Patient
- Cystic Fibrosis Lung
- Cystic Fibrosis Airway
Genome Medicine
ISSN: 1756-994X
- Submission enquiries: [email protected]
- General enquiries: [email protected]
We have a new app!
Take the Access library with you wherever you go—easy access to books, videos, images, podcasts, personalized features, and more.
Download the Access App here: iOS and Android . Learn more here!
- Remote Access
- Save figures into PowerPoint
- Download tables as PDFs

Chapter 19: Case Study: Cystic Fibrosis
Julie M. Skrzat; Carole A. Tucker
- Download Chapter PDF
Disclaimer: These citations have been automatically generated based on the information we have and it may not be 100% accurate. Please consult the latest official manual style if you have any questions regarding the format accuracy.
Download citation file:
- Search Book
Jump to a Section
Introduction.
- Examination: Age 2 Months
- Evaluation, Diagnosis, and Prognosis
- Intervention
- Conclusion of Care
- Examination: Age 8 Years
- Examination: Age 16 Years
- Recommended Readings
- Full Chapter
- Supplementary Content
C ystic fibrosis (CF) is an autosomal recessive condition affecting approximately 30,000 Americans and 70,000 people worldwide. According to the Cystic Fibrosis Foundation ( Cystic Fibrosis Foundation, 2019a ), approximately 1,000 new cases are diagnosed yearly in the United States, with a known incidence of 1 per 3,900 live births. The disease prevalence varies greatly by ethnicity, with the highest prevalence occurring in Western European descendants and within the Ashkenazi Jewish population.
The CF gene, located on chromosome 7, was first identified in 1989. The disease process is caused by a mutation to the gene that encodes for the CF transmembrane conductance regulator (CFTR) protein. This mutation alters the production, structure, and function of cyclic adenosine monophosphate (cAMP), a dependent transmembrane chloride channel carrier protein found in the exocrine mucus glands throughout the body. The mutated carrier protein is unable to transport chloride across the cell membrane, resulting in an electrolyte and charge imbalance. Diffusion of water across the cell membrane is thus impaired, resulting in the development of a viscous layer of mucus. The thick mucus obstructs the cell membranes, traps nearby bacteria, and incites a local inflammatory response. Subsequent bacterial colonization occurs at an early age and ultimately this repetitive infectious process leads to progressive inflammatory damage to the organs involved in individuals with CF.
Pop-up div Successfully Displayed
This div only appears when the trigger link is hovered over. Otherwise it is hidden from view.
Please Wait
- Publications
- Conferences & Events
- Professional Learning
- Science Standards
- Awards & Competitions
- Instructional Materials
- Free Resources
- American Rescue Plan
- For Preservice Teachers
- NCCSTS Case Collection
- Partner Jobs in Education
- Interactive eBooks+
- Digital Catalog
- Regional Product Representatives
- e-Newsletters
- Bestselling Books
- Latest Books
- Popular Book Series
- Prospective Authors
- Web Seminars
- Exhibits & Sponsorship
- Conference Reviewers
- National Conference • Denver 24
- Leaders Institute 2024
- National Conference • New Orleans 24
- Submit a Proposal
- Latest Resources
- Professional Learning Units & Courses
- For Districts
- Online Course Providers
- Schools & Districts
- College Professors & Students
- The Standards
- Teachers and Admin
- eCYBERMISSION
- Toshiba/NSTA ExploraVision
- Junior Science & Humanities Symposium
- Teaching Awards
- Climate Change
- Earth & Space Science
- New Science Teachers
- Early Childhood
- Middle School
- High School
- Postsecondary
- Informal Education
- Journal Articles
- Lesson Plans
- e-newsletters
- Science & Children
- Science Scope
- The Science Teacher
- Journal of College Sci. Teaching
- Connected Science Learning
- NSTA Reports
- Next-Gen Navigator
- Science Update
- Teacher Tip Tuesday
- Trans. Sci. Learning
MyNSTA Community
- My Collections
Maggie’s Illness
Protein Structure and Function in Cystic Fibrosis
By Michaela Gazdik Stofer
Share Start a Discussion
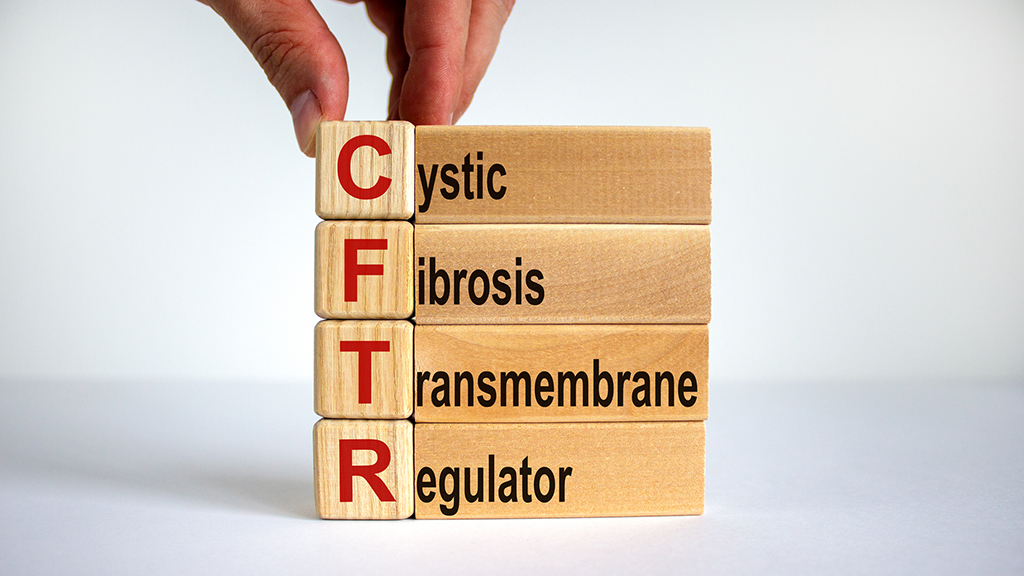
This directed case study examines the molecular basis of cystic fibrosis to emphasize the relationship between the genetic code stored in a DNA sequence and the encoded protein’s structure and function. Cystic fibrosis is caused by mutations in the cystic fibrosis transmembrane conductance regulator (CFTR) protein that functions to help maintain salt and water balance along the surface of the lung and gastrointestinal tract. This case introduces students to “Maggie,” who has just been diagnosed with cystic fibrosis. The students must identify the mutation causing Maggie’s disease by transcribing and translating a portion of the wildtype and mutated CFTR gene. Students then compare the three-dimensional structures of the resulting proteins to better understand the effect a single amino acid mutation can have on the overall shape of a protein. Students also review the concepts of tonicity and osmosis to examine how the defective CFTR protein leads to an increase in the viscosity of mucus in cystic fibrosis patients. This case was developed for use in an introductory college-level biology course but could also be adapted for use in an upper-level cell or molecular biology course.
Download Case
Date Posted
- Generate a protein sequence through transcription and translation of a given DNA gene sequence.
- Explain the chemistry of amino acid side chains and their importance in protein folding.
- Describe how a mutation in a protein sequence leads to changes in the overall tertiary structure of the protein.
- Examine various levels of protein structure using Cn3D to view three-dimensional protein structures from NCBI’s Entrez Structure database.
- Relate the loss of function of the CFTR protein to the physiological causes of cystic fibrosis.
Protein structure; transcription; translation; DNA mutation; cystic fibrosis; genetic disease; protein function; protein folding; protein; CFTR; Cn3D
Subject Headings
EDUCATIONAL LEVEL
Undergraduate lower division, Undergraduate upper division
TOPICAL AREAS
TYPE/METHODS
Teaching Notes & Answer Key
Teaching notes.
Case teaching notes are protected and access to them is limited to paid subscribed instructors. To become a paid subscriber, purchase a subscription here .
Teaching notes are intended to help teachers select and adopt a case. They typically include a summary of the case, teaching objectives, information about the intended audience, details about how the case may be taught, and a list of references and resources.
Download Notes
Answer Keys are protected and access to them is limited to paid subscribed instructors. To become a paid subscriber, purchase a subscription here .
Download Answer Key
Materials & Media
Supplemental materials.
The following two files should be viewed with the Cn3D software to view a single domain of the CFTR and ∆F508 CFTR proteins.
You may also like
Web Seminar
Join us on Tuesday, June 4, 2024, from 7:00 PM to 8:30 PM ET, to learn about the free lesson plans and storyline units designed for high school studen...
Join us on Thursday, October 24, 2024, from 7:00 PM to 8:00 PM ET, to learn about all NSTA Teacher Awards available and how to apply.Did you come up w...
Join us on Thursday, September 19, 2024, from 7:00 PM to 8:00 PM ET, to learn about NSTA election process for new and interested members of the Board ...
Thank you for visiting nature.com. You are using a browser version with limited support for CSS. To obtain the best experience, we recommend you use a more up to date browser (or turn off compatibility mode in Internet Explorer). In the meantime, to ensure continued support, we are displaying the site without styles and JavaScript.
- View all journals
- My Account Login
- Explore content
- About the journal
- Publish with us
- Sign up for alerts
- Open access
- Published: 22 March 2023
CFTR function, pathology and pharmacology at single-molecule resolution
- Jesper Levring ORCID: orcid.org/0000-0002-8916-4241 1 ,
- Daniel S. Terry 2 ,
- Zeliha Kilic 2 ,
- Gabriel Fitzgerald 3 ,
- Scott C. Blanchard ORCID: orcid.org/0000-0003-2717-9365 2 &
- Jue Chen ORCID: orcid.org/0000-0003-2075-4283 1 , 4
Nature volume 616 , pages 606–614 ( 2023 ) Cite this article
18k Accesses
14 Citations
105 Altmetric
Metrics details
- Chloride channels
- Cryoelectron microscopy
- Membrane proteins
- Single-molecule biophysics
An Author Correction to this article was published on 04 May 2023
This article has been updated
The cystic fibrosis transmembrane conductance regulator (CFTR) is an anion channel that regulates salt and fluid homeostasis across epithelial membranes 1 . Alterations in CFTR cause cystic fibrosis, a fatal disease without a cure 2 , 3 . Electrophysiological properties of CFTR have been analysed for decades 4 , 5 , 6 . The structure of CFTR, determined in two globally distinct conformations, underscores its evolutionary relationship with other ATP-binding cassette transporters. However, direct correlations between the essential functions of CFTR and extant structures are lacking at present. Here we combine ensemble functional measurements, single-molecule fluorescence resonance energy transfer, electrophysiology and kinetic simulations to show that the two nucleotide-binding domains (NBDs) of human CFTR dimerize before channel opening. CFTR exhibits an allosteric gating mechanism in which conformational changes within the NBD-dimerized channel, governed by ATP hydrolysis, regulate chloride conductance. The potentiators ivacaftor and GLPG1837 enhance channel activity by increasing pore opening while NBDs are dimerized. Disease-causing substitutions proximal (G551D) or distal (L927P) to the ATPase site both reduce the efficiency of NBD dimerization. These findings collectively enable the framing of a gating mechanism that informs on the search for more efficacious clinical therapies.
Similar content being viewed by others
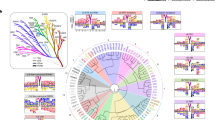
The intrinsic substrate specificity of the human tyrosine kinome
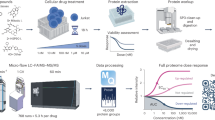
Decrypting the molecular basis of cellular drug phenotypes by dose-resolved expression proteomics
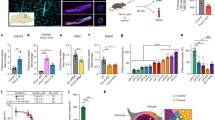
Structural and molecular basis of choline uptake into the brain by FLVCR2
CFTR belongs to the ATP-binding cassette transporter family of proteins, but uniquely functions as an ion channel 4 . It consists of two transmembrane domains that form an ion permeation pathway, two cytosolic NBDs that bind and hydrolyse ATP, and a cytosolic regulatory (R) domain that includes several phosphorylation sites. Decades of electrophysiological, biochemical and structural studies (reviewed in refs. 5 , 6 ) established that CFTR activity requires phosphorylation of the R domain by protein kinase A (PKA) 7 . Once phosphorylated, ATP binding drives pore opening. CFTR contains two functionally distinct ATP-binding sites 8 . The ‘consensus’ site is catalytically competent, whereas the ‘degenerate’ site is not 9 . ATP hydrolysis at the consensus site leads to pore closure 10 . Pore opening in the absence of ATP and non-hydrolytic pore closure can occur, albeit very rarely 11 , 12 .
Cryogenic electron microscopy (cryo-EM) studies of CFTR have thus far revealed two globally distinct conformations. In the absence of phosphorylation and ATP, CFTR forms a pore-closed conformation in which the NBDs are separated by approximately 20 Å, and the R domain sterically precludes NBD dimerization 13 (Fig. 1a ). The phosphorylated and ATP-bound CFTR, structurally characterized using the hydrolysis-deficient E1371Q variant 14 , exhibits a pre-hydrolytic conformation, in which the NBDs form a closed dimer with two ATP molecules bound at their interface (Fig. 1a ).
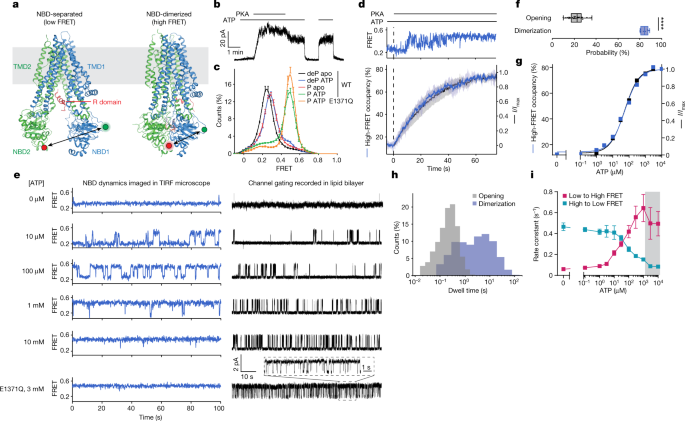
a , CFTR structures in dephosphorylated, ATP-free (left, Protein Data Bank 5UAK ) and phosphorylated, ATP-bound (right, Protein Data Bank 6MSM ) states. Green and red circles indicate fluorophore positions. b , Inside-out excised patch showing dependence of wild-type (WT) CFTR-mediated currents on phosphorylation and ATP. Concentrations of 300 nM PKA and 3 mM ATP were used. c , FRET histograms for dephosphorylated (deP) and phosphorylated (P) wild-type CFTR FRET in the presence and absence of ATP, and phosphorylated CFTR FRET (E1371Q) with ATP. Data represent means and standard errors for n independent experiments. n = 6 for wild-type dephosphorylated and phosphorylated apo, n = 5 for wild-type dephosphorylated with ATP, n = 7 for wild-type phosphorylated with ATP, and n = 3 for phosphorylated E1371Q with ATP. d , Activation of pore opening and increase in occupancy of the high-FRET state after application of 300 nM PKA (at the dashed line), in the presence of 3 mM ATP. Upper panel, representative smFRET trace of CFTR FRET during phosphorylation. Lower panel, population-wide time-dependent changes in current and high-FRET occupancy after PKA application. Data represent means and standard deviations (shaded area) for three patches and three FRET experiments. e , Sample 100-s excerpts of traces from smFRET with phosphorylated CFTR FRET (left) and single-channel electrophysiology in lipid bilayers with phosphorylated wild-type CFTR (right) at the indicated ATP concentrations. In electrophysiology traces, upward deflections correspond to opening. The bottom traces are with the E1371Q variant in 3 mM ATP. f , Probabilities of opening and dimerization of phosphorylated CFTR in 3 mM ATP. Whiskers represent minima and maxima and boxes represent 25th, 50th and 75th percentiles for 39 bilayers and 8 FRET experiments. Statistical significance was tested by two-tailed Student’s t -test (**** P = 2 × 10 −18 ). g , ATP dose responses of CFTR-mediated current and high-FRET-state occupancy. Responses were fitted using the Hill equation with an EC 50 of 53 ± 4 µM for opening and an EC 50 of 55 ± 8 µM for high-FRET occupancy. Hill coefficients were fixed to 1. h , Dwell-time distributions of opening and dimerization events for phosphorylated CFTR in 3 mM ATP. i , ATP dose responses for rates of transitioning between low- and high-FRET states for phosphorylated CFTR FRET . Data represent means and standard errors for three experiments. The shaded area indicates the regime in which transitions are obscured by time averaging, resulting in erroneous rate estimates.
Despite these advances, major gaps in our understanding of CFTR function and regulation remain. For example, although extant structures of CFTR indicate that large-scale conformational changes are required for channel opening, they fall short of addressing the mechanistic relationship between NBD dimerization and the gating mechanism. How ion permeation is coupled to ATP hydrolysis and NBD isomerization remains contested. One model proposes that in every gating cycle one round of ATP hydrolysis is coupled with one pore-opening event and one NBD-dimerization and NBD-separation event 13 , 15 , 16 , 17 . Alternative models posit that the NBDs remain dimerized through several gating cycles with only partial disengagement of the dimer interface at the consensus site 10 , 18 , 19 . The CFTR pore has been suggested to be either strictly 10 , 18 or probabilistically 19 coupled to nucleotide state in the consensus site. Attempts to differentiate these models have thus far been inconclusive. Moreover, the steps rate-limiting to CFTR activity in unaffected individuals and patients with cystic fibrosis, and thus most likely to be sensitive to pharmacological modulation, remain unclear.
To address these open questions, we undertook an integrative approach combining ensemble measurements of ATPase activity, single-molecule fluorescence resonance energy transfer (smFRET) imaging, electrophysiology and kinetic simulations to examine the structure–function relationship in human CFTR. The information obtained reveals an allosteric gating mechanism in which ATP-dependent NBD dimerization is insufficient to enable pore opening. Although phosphorylated CFTR predominantly occupies an NBD-dimerized conformation at physiological ATP concentration, downstream conformational changes in CFTR governed by ATP turnover are required for chloride conductance. Disease-associated alterations and the pharmacological potentiators ivacaftor and GLPG1837 influence the efficiency of the coupling between NBD dimerization and ion permeation. These findings identify an allosteric link between the catalytically competent ATP-binding site and the channel pore that functions as a critical rate-limiting conduit for physiological and pharmacological regulation in CFTR.
CFTR variant for smFRET retains native activity
To enable smFRET imaging of the protein’s conformational state, we sought to develop a variant of human CFTR that could be labelled with maleimide-activated donor and acceptor fluorophores. After substituting 16 of the 18 native cysteines (Extended Data Fig. 1a ), we further introduced cysteines into NBD1 (T388C) and NBD2 (S1435C). This variant, CFTR FRET , was labelled with maleimide-activated forms of self-healing donor (LD555) and acceptor (LD655) fluorophores 20 to create an NBD-dimerization sensor (Fig. 1a ). Labelling of the two introduced cysteines was >90% specific (Extended Data Fig. 2a ).
We next tested whether CFTR FRET retains the functional properties of the wild-type CFTR. Macroscopic currents were measured in excised inside-out membrane patches using unlabelled wild-type CFTR and CFTR FRET , both fused to a carboxy-terminal GFP tag (Extended Data Fig. 1b–g ). These data show that CFTR FRET conducted phosphorylation- and ATP-dependent currents and retained sensitivity to the potentiator GLPG1837 in a manner indistinguishable from that of wild-type CFTR (Extended Data Fig. 1b,c ). The time courses for current activation on ATP application and current relaxation on ATP withdrawal were also indistinguishable between wild-type CFTR and CFTR FRET (Extended Data Fig. 1d–g ).
We further evaluated the effects of conjugating fluorophores to CFTR using purified protein. Digitonin-solubilized and fluorophore-labelled CFTR FRET (Extended Data Fig. 2a,b ) hydrolysed ATP at a rate nearly identical to that of wild-type CFTR (Extended Data Fig. 1h ). On reconstitution into synthetic planar lipid bilayers, the fluorophore-labelled CFTR FRET and wild-type CFTR (without fluorophore labels) exhibited similar current–voltage relationship, open probability, and response to GLPG1837 (Extended Data Fig. 1i–m ). Single-channel conductance of fluorophore-labelled CFTR FRET was slightly higher (Extended Data Fig. 1i,j ), possibly due to the C343S substitution, a residue bordering the pore. On the basis of these observations, we conclude that the conformational and gating dynamics of CFTR FRET closely recapitulate those of wild-type CFTR.
NBD dimerization is insufficient for pore opening
To examine the relationship between ATP binding and NBD dimerization directly, we carried out smFRET imaging on digitonin-solubilized, C-terminally His-tagged CFTR FRET molecules that were surface-tethered within passivated microfluidic chambers via a streptavidin–biotin–tris-(NTA-Ni 2+ ) bridge (Extended Data Fig. 2c ). Imaging was carried out using a wide-field total internal reflection fluorescence (TIRF) microscope equipped with scientific complementary metal–oxide sensor (sCMOS) detection and stopped-flow capabilities 21 at 10 or 100 ms time resolution. Monomeric CFTR FRET molecules were tethered with high specificity as demonstrated by near-quantitative release from the surface with imidazole (Extended Data Fig. 2d–g ).
Based on extant structures, fluorophore-labelled CFTR FRET is expected to exhibit low FRET efficiency in NBD-separated conformations and higher FRET efficiency in NBD-dimerized conformations (Fig. 1a ). Indeed, in the absence of ATP and phosphorylation, CFTR exhibited a homogeneous low-FRET-efficiency distribution centred at 0.25 ± 0.01 (mean ± s.d. across six repeats) and exhibited few, if any, FRET fluctuations (Fig. 1c and Extended Data Fig. 3a ). Consistent with current increase on phosphorylation and ATP addition (Fig. 1b ), smFRET measurements also showed that adding ATP to phosphorylated CFTR caused a shift to higher FRET efficiency (0.49 ± 0.02), in which only brief excursions to lower-FRET states were evidenced (Fig. 1c and Extended Data Fig. 3d ). Substitution of the catalytic base in the consensus site (E1371Q), which prevents ATP hydrolysis, further stabilized CFTR in higher-FRET-efficiency conformations (Fig. 1c ). On the basis of these observations, we ascribed the ≈0.25 and ≈0.49 FRET states to NBD-separated and NBD-dimerized CFTR conformations evidenced by cryo-EM, respectively.
In contrast to the case for phosphorylated CFTR, addition of ATP to the dephosphorylated channel caused only a small shift in FRET efficiency, from ≈0.25 to 0.28 ± 0.01 (Fig. 1c and Extended Data Fig. 3b ). The FRET distribution of phosphorylated, ATP-free CFTR was also centred at 0.28 ± 0.02 (Fig. 1c and Extended Data Fig. 3c ). To explore the molecular basis of this shift, we determined the cryo-EM structure of the dephosphorylated wild-type CFTR in the presence of 3 mM ATP to 4.3 Å resolution (Extended Data Fig. 4 and Extended Data Table 1 ). Consistent with the smFRET data, the overall CFTR architecture was largely indistinguishable from that of the ATP-free CFTR structure. However, at both NBD1 and NBD2 binding sites, density corresponding to the ATP molecule was clearly evidenced (Extended Data Fig. 4d ). These data indicate that ATP binding to the dephosphorylated CFTR does not induce any global conformational change. The small shift in FRET efficiency is probably due to local changes that affect either the position and/or dynamics of the sites of labelling.
Consistent with the gradual increase in open probability observed for single channels 6 , pre-steady-state measurements of PKA-mediated CFTR phosphorylation in the presence of saturating ATP (3 mM) revealed that individual CFTR FRET molecules did not always instantaneously transition to a stably NBD-dimerized state (Fig. 1d and Extended Data Fig. 3m ). Instead, stable NBD dimerization was achieved through processes that involved rapid sampling of NBD-separated and NBD-dimerized states. Parallel electrophysiological recordings revealed matching progression of current activation (Fig. 1d ). NBD dimerization was fully reversible by phosphatase treatment (Extended Data Fig. 3n,p,r ). By contrast, the E1371Q substitution slowed NBD separation (Extended Data Fig. 3o,q–r ), indicating that ATP turnover facilitates NBD separation. These observations suggest that the gradual transition to steady-state channel activation probably reflects stochastic ATP binding to the individual NBDs and/or transient reinsertion of partially phosphorylated R domain, which resolve to stable NBD dimerization only when the R domain becomes fully phosphorylated and both NBDs are simultaneously ATP bound.
The ATP dose responses for NBD dimerization and current activation for fully phosphorylated CFTR strongly correlated, both yielding half-maximum effective concentration (EC 50 ) values of approximately 50 µM (Fig. 1e,g and Extended Data Fig. 5a,b,e ). This finding is indicative of both processes being limited by the same underlying molecular event. NBD dimerization and channel-open probabilities differed substantially: at saturating ATP concentration, approximately 85% of CFTR FRET molecules were in the NBD-dimerized conformation but the channel-open probability was only 22% (Fig. 1f ). We thus conclude that both conductive and non-conductive NBD-dimerized states must exist.
Consistent with this notion, the observed FRET dynamics differed from the evidenced gating dynamics (Fig. 1e,h ). The rate of CFTR pore opening exhibits a saturable dependence on ATP concentration whereas the channel closing rate remains constant 16 , 22 . By contrast, NBD-dimerization and NBD-separation rates both changed monotonically with ATP concentration (Fig. 1i ). At saturating ATP concentration, the dwell time of the NBD-dimerized state was approximately 20 times longer than that of the channel-open state (Fig. 1h ), suggesting that FRET-silent processes occur within the NBD-dimerized conformation that trigger channel opening and closure and that only subtle rearrangements at the dimer interface are required for nucleotide exchange. This conclusion was supported by analogous imaging studies carried out at both 10 and 100 ms time resolutions (Extended Data Fig. 3e ). We conclude that CFTR remains stably dimerized through multiple gating cycles or that transitions to partially separated NBD states are either FRET silent or occur on timescales markedly exceeding the temporal resolution of our measurements (100 s −1 ). Both models nonetheless specify that NBD dimerization is not strictly coupled to channel opening.
At a cellular ATP to ADP ratio (≈10:1), fully phosphorylated CFTR FRET predominantly occupied dimerized conformations (Extended Data Fig. 3l ), in line with CFTR predominantly binding ATP in the physiological setting. High ADP concentrations were, however, able to competitively inhibit both NBD dimerization and channel opening 23 , 24 (Extended Data Fig. 3f–l ).
To validate the physiological relevance of these findings, we carried out targeted smFRET imaging studies with phosphorylated CFTR FRET reconstituted into proteoliposomes (Extended Data Fig. 6a ). In the absence of ATP, membrane-embedded CFTR FRET molecules stably occupied the NBD-separated (0.28) FRET state (Extended Data Fig. 6b ). On addition of 3 mM ATP, CFTR FRET molecules transitioned to the NBD-dimerized (0.49) FRET state (Extended Data Fig. 6c ). The fraction of ATP-responsive molecules was reduced, probably due to degradations in channel activity or mixed orientations in the bilayer. However, the molecules that responded predominantly occupied NBD-dimerized conformations at steady state, with only rare, transient excursions to states with low FRET efficiency (Extended Data Fig. 6d ). Also consistent with expectation, CFTR FRET molecules relaxed to the NBD-separated state on ATP withdrawal (Extended Data Fig. 6e,f ). These observations demonstrate that physical properties of the digitonin-solubilized CFTR FRET recapitulate those present in the lipid bilayer. To ensure the most robust signals and statistics, we carried out the remainder of our smFRET experiments using digitonin-solubilized CFTR FRET .
The ATP-binding sites contribute asymmetrically
In CFTR, the consensus ATP-binding site hydrolyses approximately 0.3 to 1 ATP molecules per second, whereas the degenerate site retains ATP for minutes 18 , 25 . We reasoned that ATP binding in the degenerate site alone is sufficient for NBD dimerization, whereas ATP binding in the consensus site is required for channel opening. To test this hypothesis, we sought to deconvolute the individual contributions of the two ATP-binding sites by substituting aromatic ATP-stacking residues, W401 and Y1219 (ref. 26 ), with alanine to reduce the affinity for ATP at the degenerate and consensus sites, respectively (Fig. 2a ). Whereas the degenerate site variant W401A hydrolysed ATP at a rate comparable to that of the wild-type CFTR, the ATPase activity of the consensus site variant Y1219A only marginally exceeded the background, established by analogous measurements of the E1371Q variant (Fig. 2b ). The activity of the double variant (Y1219A/W401A) was indistinguishable from that of the Y1219A variant (Fig. 2b ). These data show that the Y1219A substitution nearly abolished functionally relevant ATP-binding events at the consensus site.
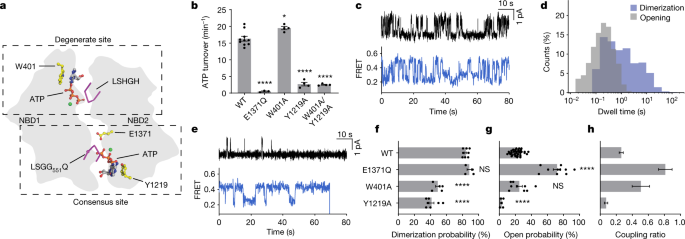
a , Schematic of degenerate and consensus sites as viewed from the plasma membrane. b , Steady-state ATP hydrolysis rates for the wild-type CFTR and variants. Data represent means and standard errors for 10 (wild-type), 3 (E1371Q) or 4 (W401A, Y1219 and W401A/Y1219A) measurements. * P = 0.014; **** P = 1.2 × 10 −11 (E1371Q), 2.7 × 10 −11 (Y1219A) and 2.1 × 10 −11 (W401A/Y1219A). c , Sample traces from single-channel electrophysiology (top) and smFRET (bottom) of the CFTR(W401A) variant. The substitution was made in wild-type CFTR and CFTR FRET backgrounds for electrophysiology and smFRET, respectively. In electrophysiology traces, upward deflections correspond to opening. d , Dwell-time distributions of opening and dimerization events for CFTR(W401A). e , As in c , but with the CFTR(Y1219A) variant. f , Dimerization probabilities of wild-type CFTR FRET and variants. Data represent means and standard errors for 8 (wild-type), 4 (E1371Q), 5 (W401A) and 7 (Y1219A) measurements. NS, not significant; **** P = 8.0 × 10 −9 (W401A) and 4.4 × 10 −11 (Y1219A). g , Open probabilities of CFTR variants. Data represent means and standard errors for 39 (wild-type), 10 (E1371Q), 9 (W401A) and 5 (Y1291A) bilayers. **** P = 10 −15 (E1371Q) and 4.9 × 10 −5 (Y1219A). h , Coupling ratios of CFTR variants, defined as open probability divided by dimerization probability. Data represent means and standard errors. Phosphorylated CFTR variants at 3 mM ATP were used in all panels. For relevant panels, statistical significance relative to the wild-type was tested by one-way analysis of variance.
The conformational dynamics of the W401A and Y1219A variants were markedly different, both from each other, and from those wild-type CFTR FRET (compare Fig. 1e with Fig. 2c,e ). Relative to wild-type CFTR, the W401A variant, which is capable of binding and hydrolysing ATP at the consensus site, underwent comparatively rapid transitions between NBD-separated and NBD-dimerized states that more closely resembled the dynamics of pore opening measured in electrophysiological recordings (Fig. 2c ). This was predominantly attributed to a specific reduction in the dwell time of the NBD-dimerized state (compare Fig. 1h with Fig. 2d ). By contrast, the Y1219A variant, which binds ATP principally at the degenerate site, slowly transitioned between NBD-dimerized and NBD-separated states (Fig. 2e ). Whereas NBD dimerization and channel-open probabilities became more comparable in the W401A variant (Fig. 2c,d ), single-channel measurements of the Y1219A variant exhibited only sporadic opening events (Fig. 2e ). These findings indicate that NBD dimerization is largely uncoupled from channel gating when ATP binding and hydrolysis is abrogated at the consensus site.
At 3 mM ATP, the dimerization probabilities of the W401A and Y1219A variants were comparable, at about 50% of the wild-type level (Fig. 2f ). The channel-open probabilities of the two variants were, however, very different (Fig. 2g ). Whereas the W401A variant functioned like wild-type CFTR in this regard, the open probability of the Y1219A variant was nearly zero. These data indicate that ATP binding at either degenerate or consensus sites is sufficient for NBD dimerization. They further support that transitions to NBD-dimerized states do not necessarily precipitate ATP hydrolysis or channel opening and that channel opening largely depends on ATP binding to the consensus site.
These conclusions were further substantiated through assessment of the ‘coupling ratio’ between open probability and the probability of NBD dimerization (Fig. 2h ), which showed that the coupling efficiency was far more sensitive to occupancy of the consensus site by ATP. The coupling ratio of the W401A variant was sixfold greater than that of the Y1219A variant. The extent of coupling between NBD dimerization and channel opening was the greatest for the E1371Q variant, which traps the pre-hydrolytic NBD-dimerized state with both sites occupied by ATP (Fig. 1e ).
NBD dimerization precedes channel opening
To examine the temporal relationship between ATP-dependent NBD dimerization and channel opening, we carried out parallel experiments in which the pre-steady state of smFRET and electrophysiological CFTR reaction coordinates were monitored in response to rapid ATP addition (Fig. 3a ). Here we separately tracked the time courses of NBD dimerization and macroscopic current increase on application of saturating ATP (3 mM) to CFTR FRET , which had been previously phosphorylated by PKA treatment, followed by complete ATP removal from the system.
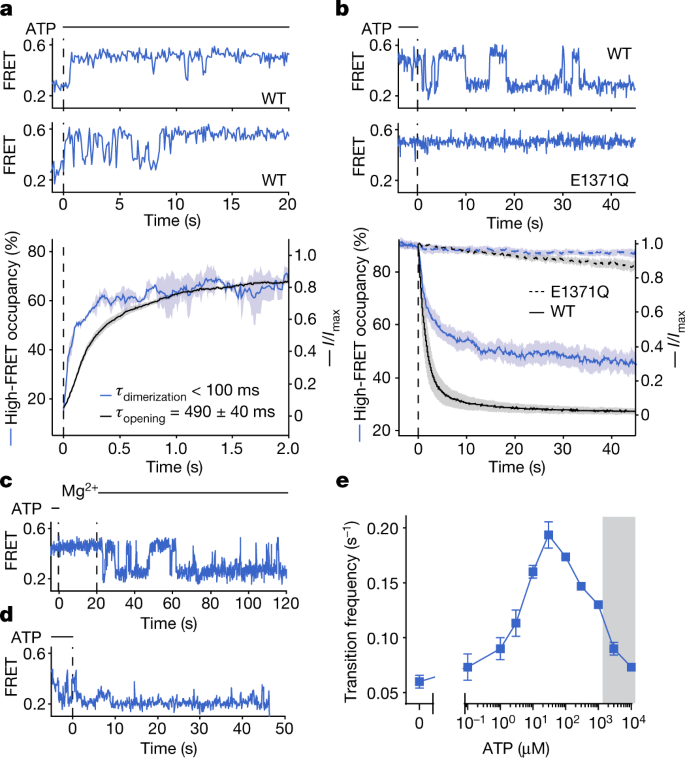
a , Upper panels, representative smFRET traces of ATP delivery (at the dashed line) to phosphorylated and nucleotide-free wild-type CFTR FRET . Lower panel, time-dependent changes in high-FRET occupancy of CFTR FRET and wild-type CFTR current after ATP delivery. Data represent means (solid line) and standard errors (shaded area) of 3 FRET experiments and 42 patches. Individual time courses were fitted as mono-exponential relaxations (see Extended Data Fig. 7a,b ). Means and standard errors of exponential time constants are reported. b , Upper panels, representative smFRET traces of ATP withdrawal (at the vertical dashed line) from phosphorylated wild-type CFTR FRET and the CFTR FRET (E1371Q) variant. Lower panel, time-dependent changes in high-FRET occupancy of CFTR FRET and CFTR current after ATP withdrawal from the wild-type (solid lines) and the E1371Q (dashed lines) variant. Data represent means (line) and standard errors (shaded area) of 5 FRET experiments and 41 (wild-type) or 6 (E1371Q) patches. c , Representative single-molecule trace of ATP withdrawal from wild-type CFTR FRET . Initially Mg 2+ is absent, followed by reintroduction of 2 mM Mg 2+ . d , Representative single-molecule trace of ATP withdrawal from the CFTR FRET (W401A) variant. e , ATP dose response for the frequency of transition between low- and high-FRET states for phosphorylated wild-type CFTR FRET . Data represent means and standard errors for three experiments. The shaded area indicates the regime in which transitions are obscured by time averaging, resulting in erroneous rate estimates. ATP was used at 3 mM in all panels.
In these experiments, individual CFTR FRET molecules transitioned either directly to a stably NBD-dimerized state, or through a highly dynamic interval with rapid NBD-isomerization events (Fig. 3a ), resembling the W401A variant at steady state (Fig. 2c ). Given that no change in R domain phosphorylation occurs in this experiment, we conclude that the observed heterogeneity in NBD-dimerization kinetics reflects stochastic and sequential ATP binding to the individual NBDs, which ultimately equilibrate to both NBDs being occupied by ATP.
Comparison of the activation time courses of both reaction coordinates further revealed that channel opening on ATP binding was delayed relative to NBD dimerization (Fig. 3a ). The rate of channel opening ( τ opening = 490 ± 40 ms; Fig. 3a and Extended Data Fig. 7a ) was approximately threefold slower than the solvent exchange rate of the perfusion system ( τ exchange ≈ 150 ms; Extended Data Fig. 7c,d ). By contrast, the fitted rates for NBD dimerization from FRET measurements ( τ dimerization ≈ 100 ms) were on the same scale as the solvent exchange rate in the fluorescence microscope ( τ exchange = 115 ms; Extended Data Fig. 7b,e ). Thus, the observed delay in current activation could not be ascribed to differences in rates of mixing of the two experimental methods. We therefore conclude that the observed delay reflects conformational changes within the NBD-dimerized state that precede channel opening and that the mean first-passage time of this process is approximately 400–500 ms.
Dimerization persists through cycles of hydrolysis
To understand the molecular events surrounding the process of pore closure, we monitored conformational changes and macroscopic current decays of fully phosphorylated CFTR on sudden ATP withdrawal (Fig. 3b and Extended Data Fig. 7f–l ). Consistent with the findings of previous studies 15 , 27 , our observations showed that ATP removal leads to rapid current decay that is dependent on ATP hydrolysis (Extended Data Fig. 7f,g ). Parallel FRET experiments showed that the time course of NBD separation is biphasic, with time constants of 1.6 s and 20 s, respectively (Fig. 3b ). These rates correlate with the double-exponential time constants reported for CFTR current decay and ligand exchange 18 , 28 . This apparent correlation suggests a common underlying molecular mechanism determining both transitions.
Inhibiting ATP hydrolysis with the E1371Q substitution markedly slowed NBD separation, and biochemical approaches to reduce ATP hydrolysis or conformational events immediately following hydrolysis—including magnesium withdrawal, as well as beryllium fluoride or aluminium fluoride addition—also resulted in much slower NBD separation (Fig. 3b,c and Extended Data Fig. 7i,j ).
On ATP withdrawal, individual CFTR FRET molecules first exhibited dynamic NBD isomerization, followed by stable NBD separation (Fig. 3b ). This dynamic period resembled the steady-state behaviour of the Y1219A variant (Fig. 2e ). Disruption of ATP binding at the degenerate site by the W401A substitution eliminated this dynamic period, such that transitions occurred directly from the NBD-dimerized state to the NBD-separated state on ATP withdrawal (Fig. 3d and Extended Data Fig. 7h,k ). These observations suggest that the dynamic period represents a post-hydrolytic state, in which the consensus site becomes vacated, and the degenerate site retains ATP. As ATP rebinding is not possible in this experiment, subsequent ATP dissociation from the degenerate site then precipitates stable NBD separation (Extended Data Fig. 7l ). Dissociation of ATP from both sites probably leads to the reversible rundown of CFTR currents that occurs after prolonged exposure to nucleotide-free solutions 29 .
ATP rebinding at physiological ATP concentrations (approximately 1–10 mM) is expected to occur rapidly to the post-hydrolytic CFTR molecule, thereby initiating new catalytic cycles before NBD separation. Consistent with this notion, the transition frequency between the low- and high-FRET states exhibited a bell-shaped dependence on ATP concentration (Fig. 3e and Extended Data Fig. 5f ). These findings suggest that the probability of ATP rebinding exceeds that of complete NBD separation when ATP concentration is greater than 100 µM. This concept, consistent with ligand exchange experiments 18 , suggests that repetitive cycles of ATP turnover can occur in an ostensibly NBD-dimerized conformation with only subtle changes at the consensus site required for nucleotide exchange. In cellular settings, repetitive gating cycles are therefore expected to persist until the finite rate of NBD separation at cellular ATP concentrations allows the dephosphorylated R domain to reinsert, terminating CFTR gating.
Disease mutations disrupt allosteric coupling
A wide range of alterations in CFTR are directly linked to cystic fibrosis 30 (Extended Data Fig. 8a ). The mechanisms by which such alterations affect an individual’s health have been broadly categorized into those that interfere with CFTR expression, folding or localization or the function of the channels on the cell surface ( https://www.cftr2.org/mutations_history ). Here we examine two clinically evidenced variants that affect channel gating at the cell surface, G551D and L927P, to understand the molecular basis of their defects.
The G551 residue forms part of the consensus ATP-binding site that coordinates the phosphate moieties for hydrolysis 31 (Figs. 2a and 4a ). Substituting G551 with an aspartate nearly abolished channel opening 32 and ATP hydrolysis (Fig. 4b–d ). On addition of saturating ATP (3 mM) to the phosphorylated CFTR FRET (G551D) variant, we observed an upward shift in FRET efficiency from the NBD-separated state (≈0.25 FRET efficiency) to 0.37 ± 0.01. This intermediate FRET efficiency value was clearly distinct from that of the NBD-dimerized conformation (≈0.49 FRET) observed for wild-type CFTR FRET , indicative of a conformation involving an intermediate approach of the NBDs (Fig. 4b and Extended Data Fig. 8b,d ). From this intermediate conformation, excursions to the 0.49 FRET efficiency states were evidenced, albeit rarely (Fig. 4b ). The high-FRET, NBD-dimerized CFTR(G551D) conformation is likely to be different from that of CFTR(E1371Q) previously observed by cryo-EM, evident by a lower coupling ratio (Fig. 4e ) and a shorter lifetime (Extended Data Fig. 8e,f ). In agreement with these data, the findings of a recent cryo-EM study showed that the G551D variant adopts conformations in between those of the fully NBD-separated and NBD-dimerized conformations 33 .
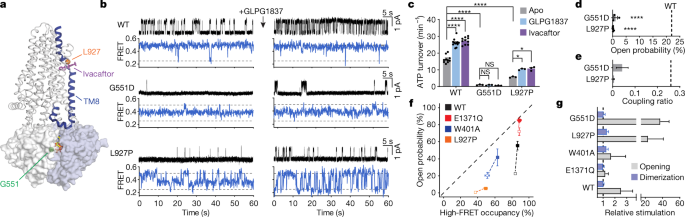
a , Cartoon representation of CFTR, indicating the positions of residues G551 and L927, and the ivacaftor-binding site. The structural link between the potentiator-binding site and the NBD interface through TM8 is coloured blue. The consensus-site ATP is shown as sticks. b , Sample traces from single-channel electrophysiology (top) and smFRET (bottom) of wild-type CFTR and the CFTR(G551D) and CFTR(L927P) variants in the absence or presence of 10 µM GLPG1837. Substitutions were made in wild-type CFTR and CFTR FRET backgrounds for electrophysiology and smFRET experiments, respectively. Horizontal dashed lines indicate mean FRET efficiencies of low- and high-FRET states. c , Steady-state ATP hydrolysis rates for the wild-type CFTR and the CFTR(G551D) and CFTR(L927P) variants. Measurements were carried out in the absence or presence of 10 µM GLPG1837 or 1 µM ivacaftor. Data represent means and standard errors for 10 (wild-type apo and with ivacaftor), 12 (wild-type with GLPG1837) and 3 (G551D and L927P all conditions) measurements. * P = 0.032 (L927P apo versus GLPG1837) and 0.017 (L927P apo versus ivacaftor); **** P = 3 × 10 −14 (wild-type apo versus GLPG1837), 4 × 10 −15 (wild-type apo versus ivacaftor), 2 × 10 −14 (wild-type versus G551D) and 8.8 × 10 −10 (wild-type versus L927P). d , Open probabilities of G551D and L927P variants. Data represent means and standard errors for 3 (G551D) or 6 (L927P) bilayers. The dashed line indicates mean open probability of wild-type CFTR. **** P = 3.1 × 10 −7 (G551D) and 4.3 × 10 −11 (L927P). e , Coupling ratios of G551D and L927P variants. Data represent means and standard errors. The dashed line indicates the coupling ratio for wild-type CFTR. f , Correlation of probabilities of dimerization and opening for wild-type CFTR, CFTR(E1371Q), CFTR(W401A) and CFTR(L927P) in the absence (open squares) or presence (filled squares) of 10 µM GLPG1837. Data represent means and standard errors for 39 (wild-type apo), 9 (wild-type GLPG1837 and W401A apo), 10 (E1371Q apo), 6 (E1371Q GLPG1837 and L927P apo), 5 (W401A GLPG1837) and 3 (L927P GLPG1837) open-probability measurements and for 8 (wild-type apo), 4 (wild-type GLPG1837, E1371Q apo and E1371Q GLP1837), 5 (W401A apo and L927P apo) and 3 (W401A GLPG1837 and L927P GLPG1837) FRET measurements. The dashed line indicates equality between probabilities of opening and dimerization. g , Relative stimulation of opening and dimerization probabilities with 10 µM GLPG1837. Data represent means and standard deviations. The dashed line indicates no stimulation. Relative stimulation of G551D opening was determined by macroscopic current measurements in excised inside-out patches. Phosphorylated CFTR variants at 3 mM ATP were used in all panels. For relevant panels, statistical significance was tested by one-way analysis of variance.
The L927 residue in CFTR resides within a transmembrane hinge region that mediates local conformational changes during gating 34 (Fig. 4a ). Thus, it would be reasonable to propose that L927P causes cystic fibrosis by altering the flexibility of the transmembrane hinge in NBD-dimerized CFTR conformations. Compared to the wild-type CFTR, the L927P substitution resulted in a 65% reduction of the ATPase activity and a >99% reduction in channel-open probability (Fig. 4c,d ). Its open dwell time was reduced by approximately 15-fold (Extended Data Fig. 8g,h ). Notably, the L927P substitution, although 50 Å away from either ATP-binding site, was also detrimental to the NBD-dimerization process. In the absence of ATP, the fully phosphorylated L927P variant behaved similarly to wild-type CFTR FRET with the NBDs constitutively separated (Extended Data Fig. 8c,d ). However, on ATP introduction (3 mM), the L927P variant adopted a conformation exhibiting an intermediate extent of NBD closure (FRET efficiency = 0.31 ± 0.01) from which relatively frequent, although transient, NBD-dimerization events occurred (Fig. 4b and Extended Data Fig. 8c–f ).
For both G551D and L927P variants, FRET transitions exhibited ATP dependence indicative of wild-type ATP binding affinities (Extended Data Fig. 8i–k ). Their functional defects are caused by deficits in ATP effecting formation of a tight NBD dimer and in the coupling of the allosteric processes within NBD-dimerized CFTR that give rise to channel opening (Fig. 4e ). On the basis of the positions of the G551D and L927P substitutions, we posit that an allosteric link transmits conformational information from the consensus ATP-binding site at the NBD dimer interface through transmembrane helix 8 (TM8) to the gating region on the opposing side of the membrane (Fig. 4a ). Distant G551D and L927P substitutions both impact this pathway of allosteric communication, indicating that local disruptions at either end of this link can affect both NBD dimerization and channel opening.
Potentiators promote opening of dimerized channels
Ivacaftor, a drug approved by the US Food and Drug Administration, and the investigational compound GLPG1837 (ref. 35 , 36 ) both bind to CFTR within the TM8 hinge region to promote channel opening 37 (Fig. 4a ). Although the effects of these compounds on gating kinetics have been extensively characterized 38 , 39 , 40 , 41 , how they alter the conformational landscape of CFTR remains elusive.
Consistent with the findings of previous reports 38 , 39 , 40 , 41 , our observations showed that both potentiators induced marked increases of channel-open probabilities (Fig. 4b,f and Extended Data Fig. 9a ). By comparison their effects on NBD dimerization were much smaller for all CFTR variants tested (Fig. 4b,f and Extended Data Fig. 9a ). For example, GLPG1837 increased the open probability of the G551D variant by more than 30-fold, whereas the change in NBD dimerization was marginal (Fig. 4b,g ). This observation, together with the recent cryo-EM study of the CFTR(G551D) variant in the presence of ivacaftor 33 , demonstrates that neither ivacaftor nor GLPG1837 promotes NBD dimerization. Similarly, for the L927P variant, the relative stimulation of open probability greatly exceeded the relative stimulation of dimerization probability (Fig. 4f,g ).
The potency with which GLPG1837 promoted NBD dimerization, measured at the EC 50 for ATP, was approximately 60 nM (Extended Data Fig. 9b,c ), similar to the estimated affinity from electrophysiology 37 . The apparent potency of ATP to mediate dimerization also increased in a dose-dependent manner with GLPG1837 (Extended Data Fig. 9d ). Furthermore, the rate of NBD separation on ATP withdrawal was slowed by GLPG1837 or ivacaftor (Extended Data Fig. 9e ), analogous to their impacts on the rate of current relaxation after ATP withdrawal 38 .
Potentiators both shorten the closed dwell time and extend the open dwell time of the pore 39 . Here we show that the steady-state ATP hydrolysis rates of wild-type CFTR and CFTR(L927P), for which hydrolysis rates were measurable, were increased by 60–100% by ivacaftor or GLPG1837 (Fig. 4c ). Hence, both potentiators exert, by opposing effects on the open and closed dwell times, a net increased flux through the gating cycle by targeting the allosteric pathway linking the NBDs to the channel gate.
These data lead to the conclusion that the main effect of ivacaftor or GLPG1837 is not to support transition from NBD-separated to NBD-dimerized conformations. Rather, these potentiators principally operate by promoting pore opening when NBDs are already dimerized. In other words, potentiators affect the coupling efficiency between NBD dimerization and channel opening, possibly by stabilizing the transmembrane domains in the pore-open configuration 41 . This effect also manifests in variants unable to form a canonical NBD dimer, such as G551D and a variant devoid of the entire NBD2 (ref. 38 ).
It has long been debated whether NBD dimerization in CFTR is strictly coupled to pore opening 5 , 6 . By directly comparing the kinetics of NBD isomerization and channel gating, we show that NBD dimerization and ion permeation are not strictly coupled but instead probabilistically linked through allosteric control mechanisms. At physiological ATP concentrations, fully phosphorylated CFTR remains NBD-dimerized for many cycles of ATP turnover and pore opening. The structure of the NBD-dimerized CFTR 14 suggests that only small changes at the consensus site, such as disrupting the hydrogen bond between R555 and T1246 (ref. 15 ), would be sufficient for nucleotide exchange. Notably, the allosteric relationship evidenced between NBD dimerization and pore opening held true across diverse conditions and CFTR variants and was sensitive to both nucleotide state in the consensus site and potentiator binding within the membrane more than 50 Å away.
These findings reveal an allosteric pathway linking the consensus ATPase site, through TM8 and the potentiator-binding site, to the gate of the pore on the opposing membrane surface. Structurally, we speculate that this pathway of long-distance information transfer minimally consists of TM8 and TM9 and the transverse alpha helix between them (Fig. 4a ). The transmission of structural information along this allosteric pathway physically linking NBD dimerization to pore opening is rate-limiting to CFTR function. Substitutions causing cystic fibrosis (for example, G551D and L927P) attenuate the strength of this allosteric pathway whereas the potentiators ivacaftor and GLPG1837 enhance it. The observation that both G551D and L927P variants are also defective in NBD dimerization suggests that modulators that quantitatively rescue this defect should work additively with ivacaftor and GLPG1837. The investigational compound 5-nitro-2-(3-phenylpropylamino) benzoate was proposed to stimulate pore opening by such a mechanism 42 .
The data presented herein, in conjunction with the vast body of literature in the field, permit us to propose a model that describes the main events accompanying the wild-type CFTR gating cycle at physiological ATP concentrations (Fig. 5 ). Dephosphorylated CFTR adopts an NBD-separated, auto-inhibited conformation as observed by cryo-EM 13 . Following phosphorylation of the R domain, the NBDs can dimerize rapidly with ATP bound at both sites (step 1 in Fig. 5 ). Rate-limiting conformational changes within CFTR that allosterically communicate information from the consensus ATP-binding site across the lipid bilayer can subsequently open the pore (step 2 in Fig. 5 ) and enable ATP hydrolysis (step 3 in Fig. 5 ). Before ATP hydrolysis, pore opening is transient, and flicker-closed states are rapidly sampled 43 . The post-hydrolytic channel, with ADP and inorganic phosphate bound at the consensus site, remains open but eventually relaxes to a non-conductive dimerized state (step 4 in Fig. 5 ). Dissociation of ADP (step 5 in Fig. 5 ) results in a dynamic intermediate to which ATP can rebind (steps 6–8 in Fig. 5 ) thereby initiating another gating cycle. Rare events are not depicted in this model as their fractional contributions are expected to be low at physiological ATP concentration. These events include release of ATP from the degenerate site 25 , 26 , and channel opening with ATP at only one site 32 , 44 , 45 or in the complete absence of nucleotide 11 , 12 , 32 , 46 .
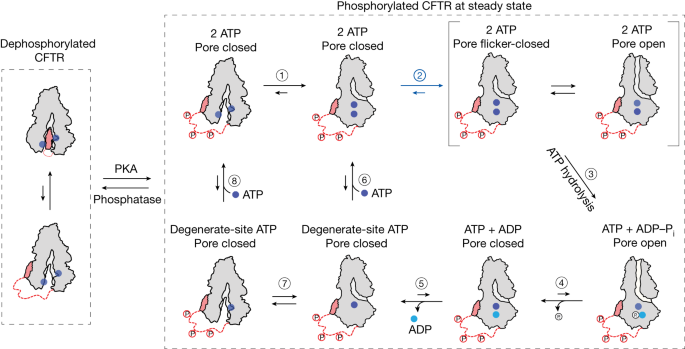
Model of the wild-type CFTR gating cycle at physiological ATP concentration. Dephosphorylated CFTR adopts an NBD-separated, auto-inhibited conformation. At steady state, the NBDs of fully phosphorylated CFTR dimerize rapidly with ATP bound at both sites (step 1). Dimerization is followed by conformational change to enable pore opening (step 2) and ATP hydrolysis at the consensus site (step 3). During the pre-hydrolytic open burst, CFTR rapidly samples a flicker-closed state. Post-hydrolytic CFTR remains open, but eventually relaxes to a non-conductive dimerized state (step 4). ADP dissociation (step 5) leads to a dynamically isomerizing intermediate (step 7). ATP rebinding may occur with subtle rearrangement at the dimer interface (step 6) or with complete NBD separation (step 8) to initiate a new gating cycle.
The topology of this scheme was validated through steady-state kinetic simulations of NBD dynamics and ion conduction for fully phosphorylated CFTR at saturating ATP concentrations (Extended Data Fig. 10 and Supplementary Methods ). Kinetic constants within this topology were estimated on the basis of the model’s capacity to recapitulate experimental observables including pre-steady-state rates of NBD dimerization, channel current and conformational relaxation as well as ATP hydrolysis rates from ensemble measurements (Extended Data Fig. 10a ). Stochastic simulations for wild-type CFTR and CFTR(E1371Q) gating carried out with this topology and rate information (Extended Data Fig. 10b,c ) closely recapitulated the key dynamical features of both wild-type and E1371Q variant gating (Extended Data Fig. 10d–h and Supplementary Video 1 ). However, establishment of this model revealed notable quantitative discrepancies that are worthy of consideration. First, our simulation predicts a modestly greater steady-state ATP hydrolysis rate than is experimentally estimated. We speculate that this may reflect either inadequacies of the simplified model or the presence of an inactive fraction in the bulk measurement that lowers the apparent turnover rate. Second, the simulated model does not recapitulate the multimodality of NBD-dimerized and NBD-separated dwell-time distributions. Such findings, viewed in light of our analyses of altered degenerate and consensus binding sites, suggest that these distinct modes probably reflect periods of NBD dynamics and gating in CFTR in which only one of the two ATP-binding sites is occupied. Such considerations imply requirements for additional complexities to the presented model topology that will need to be explored by developing techniques that simultaneously detect conformational state and functional output at single-molecule resolution. The present investigations nonetheless reveal a physical framework for understanding CFTR function, pathology and pharmacology and exploring the possibility that more potent activators of the rate-limiting allosteric events regulating CFTR gating mechanism, potentially specific to an individual’s allelic variation, can be identified and leveraged for therapeutic purposes.
Protein expression, purification and labelling
CFTR was expressed as previously described 47 . Human CFTR with a C-terminal PreScission Protease-cleavable GFP tag was cloned into the BacMam vector. For single-molecule FRET, the following substitutions were introduced: C76L, C128S, C225S, C276S, C343S, T388C, C491S, C592M, C647S, C832S, C866S, C1344S, C1355S, C1395S, C1400S, C1410S, S1435C and C1458S. A deca-His tag was inserted C-terminally to CFTR and before the PreScission Protease cleavage site to allow for surface immobilization.
Recombinant baculovirus was generated using Sf9 cells (Gibco, catalogue number 11496015, lot number 1670337) cultured in sf-900 SFM medium (Gibco), supplemented with 5% (v/v) heat-inactivated fetal bovine serum and 1% (v/v) antibiotic–antimycotic (Gibco) as described previously 48 . HEK293S GnTI − (ATCC CRL-3022, lot number 62430067) suspension cells were cultured in FreeStyle 293 medium (Gibco) supplemented with 2% (v/v) heat-inactivated fetal bovine serum and 1% (v/v) antibiotic–antimycotic (Gibco), shaking at 37 °C with 8% CO 2 and 80% humidity. Sf9 and HEK293S GnTI − cells were authenticated by Gibco and ATCC, respectively and confirmed negative for mycoplasma contamination. At a density of 2.5 × 10 6 cells ml −1 , cells were infected with 10% (v/v) P3 baculovirus. After 12 h, the culture was supplemented with 10 mM sodium butyrate, and the temperature was reduced to 30 °C. After a further 48 h, the cells were collected and flash-frozen in liquid nitrogen.
For protein purification, cells were solubilized for 75 min at 4 °C in extraction buffer containing 1.25% (w/v) lauryl maltose neopentyl glycol (LMNG), 0.25% (w/v) cholesteryl hemisuccinate (CHS), 200 mM NaCl, 20 mM HEPES (pH 7.2 with NaOH), 2 mM MgCl 2 , 10 μM dithiothreitol (DTT), 20% (v/v) glycerol, 1 mM ATP, 1 μg ml −1 pepstatin A, 1 μg ml −1 leupeptin, 1 μg ml −1 aprotinin, 100 μg ml −1 soy trypsin inhibitor, 1 mM benzamidine, 1 mM phenylmethylsulfonyl fluoride (PMSF) and 3 µg ml −1 DNase I. Lysate was clarified by centrifugation at 75,000 g for 2 × 20 min at 4 °C, and mixed with NHS-activated Sepharose 4 Fast Flow resin (GE Healthcare) conjugated with GFP nanobody, which had been pre-equilibrated in 20 column volumes of extraction buffer. After 1 h, the resin was packed into a chromatography column, washed with 20 column volumes of wash buffer containing 0.06% (w/v) digitonin, 200 mM NaCl, 20 mM HEPES (pH 6.8 with NaOH), 1 mM ATP and 2 mM MgCl 2 , and then incubated for 2 h at 4 °C with 0.35 mg ml −1 PreScission Protease to cleave off the GFP tag. The eluate was collected by dripping through Glutathione Sepharose 4B resin (Cytiva) to remove PreScission Protease, and CFTR was concentrated to 2 μM.
To label with fluorophores, CFTR was mixed with 9.5 μM maleimide-conjugated LD555 and 10.5 μM maleimide-conjugated LD655 (Lumidyne Technologies) for 10 min at 4 °C. Subsequent steps were carried out protected from light. The labelling reaction was quenched by addition of 2 mM DTT, and the labelled product was purified by gel filtration chromatography at 4 °C using a Superose 6 10/300 GL column (GE Healthcare), equilibrated with 0.06% (w/v) digitonin, 200 mM NaCl, 20 mM HEPES (pH 7.2 with NaOH), 1 mM ATP and 2 mM MgCl 2 . Peak fractions were concentrated to 2 μM and mixed with 5 μM biotin–tris-NTA-Ni 2+ for 30 min at 4 °C. The CFTR–Ni-NTA complex was purified by another round of gel filtration, concentrated to 2 μM, aliquoted, snap-frozen in liquid nitrogen, and stored at –80 °C.
For ATP hydrolysis measurements, the purification protocol was adjusted: extraction buffer contained 1.25% (w/v) LMNG, 0.25% (w/v) CHS, 200 mM KCl, 20 mM HEPES (pH 8.0 with KOH), 2 mM MgCl 2 , 2 mM DTT, 20% (v/v) glycerol, 1 μg ml −1 pepstatin A, 1 μg ml −1 leupeptin, 1 μg ml −1 aprotinin, 100 μg ml −1 soy trypsin inhibitor, 1 mM benzamidine, 1 mM PMSF and 3 µg ml −1 DNase I. Wash and gel filtration buffers contained 0.06% (w/v) digitonin, 20 mM HEPES (pH 8.0 with KOH), 200 mM KCl, 2 mM MgCl 2 and 2 mM DTT. The eluate from the GFP nanobody resin was concentrated, phosphorylated with PKA (NEB) for 1 h at 25 °C, purified by gel filtration chromatography, and immediately used for hydrolysis measurements.
For proteoliposome reconstitution, the purification was also adjusted: extraction buffer contained 1.25% (w/v) LMNG, 0.25% (w/v) CHS, 200 mM NaCl, 20 mM HEPES (pH 7.2 with NaOH), 2 mM MgCl 2 , 2 mM DTT, 20% (v/v) glycerol, 1 μg ml −1 pepstatin A, 1 μg ml −1 leupeptin, 1 μg ml −1 aprotinin, 100 μg ml −1 soy trypsin inhibitor, 1 mM benzamidine, 1 mM PMSF and 3 µg ml −1 DNase I. Wash and gel filtration buffers contained 0.006% (w/v) glyco-diosgenin (GDN), 200 mM NaCl, 20 mM HEPES (pH 7.2 with NaOH) and 2 mM MgCl 2 . The eluate from the GFP nanobody resin was concentrated, phosphorylated with PKA (NEB) for 1 h at 25 °C, purified by gel filtration chromatography, and immediately reconstituted.
ATP hydrolysis measurements
Steady-state ATP hydrolysis activity was measured using an NADH-coupled assay 49 . Reaction buffer contained 50 mM HEPES (pH 8.0 with KOH), 150 mM KCl, 2 mM MgCl 2 , 2 mM DTT, 0.06% (w/v) digitonin, 60 µg ml −1 pyruvate kinase (Roche), 32 µg ml −1 lactate dehydrogenase (Roche), 9 mM phosphoenolpyruvate and 150 µM NADH, and was prepared immediately before starting the assay. A 200 nM concentration of phosphorylated CFTR was diluted into reaction buffer. Aliquots of 30 µl in volume were distributed into a Corning 384-well Black/Clear Flat Bottom Polystyrene NBS Microplate. Samples were kept at 4 °C and light-protected until the reactions were initiated by addition of 3 mM ATP. The rate of fluorescence depletion was monitored at λ ex = 340 nm and λ em = 445 nm at 28 °C with an Infinite M1000 microplate reader (Tecan), and converted to ATP turnover with an NADH standard curve.
Patch-clamp recording
Chinese hamster ovary cells (ATCC CCL-61, lot number 70014310) were maintained in DMEM-F12 (ATCC) supplemented with 10% (v/v) heat-inactivated fetal bovine serum and 1% (v/v) GlutaMAX (Gibco) at 37 °C. Chinese hamster ovary cells were authenticated by ATCC. The cells were plated in 35-mm cell culture dishes (Falcon) 24 h before transfection. Cells were transfected with C-terminally GFP-fused CFTR cloned into the BacMam expression vector, using Lipofectamine 3000 according to the manufacturer’s protocol (Invitrogen). At 12 h following transfection, medium was replaced with DMEM-F12 supplemented with 2% (v/v) heat-inactivated fetal bovine serum and 1% (v/v) GlutaMAX, and the cells were then incubated for 24 h at 30 °C before recording.
Bath solution contained 145 mM NaCl, 2 mM MgCl 2 , 5 mM KCl, 1 mM CaCl 2 , 5 mM glucose, 5 mM HEPES and 20 mM sucrose (pH 7.4 with NaOH). Pipette solution contained 140 mM NMDG, 5 mM CaCl 2 , 2 mM MgCl 2 and 10 mM HEPES (pH 7.4 with HCl). Perfusion solution contained 150 mM NMDG, 2 mM MgCl 2 , 1 mM CaCl 2 , 10 mM EGTA and 8 mM Tris (pH 7.4 with HCl). Magnesium was omitted where indicated. CFTR was activated by exposure to PKA (Sigma-Aldrich) and 3 mM ATP.
The rate of buffer exchange by the perfusion system was estimated by exchanging perfusion solution with 150 mM NMDG, 2 mM MgSO 4 , 1 mM calcium gluconate, 10 mM EGTA and 8 mM Tris (pH 7.4 with H 2 SO 4 ).
Pipettes were pulled from borosilicate glass (outer diameter 1.5 mm, inner diameter 0.86 mm, Sutter) to 1.5–2.5 MΩ resistance and fire polished. Recordings were carried out using the inside-out patch configuration with local perfusion at the patch. Membrane potential was clamped at –30 mV. Currents were recorded at 25 °C using an Axopatch 200B amplifier, a Digidata 1550 digitizer and the pClamp software suite (Molecular Devices). Recordings were low-pass-filtered at 1 kHz and digitized at 20 kHz. All displayed recordings were further low-pass filtered at 100 Hz. Data were analysed with Clampfit, GraphPad Prism and OriginPro.
Proteoliposome reconstitution
A lipid mixture containing 1,2-dioleoyl- sn -glycero-3-phosphoetanolamine, 1-palmitoyl-2-oleyl- sn -glycero-3-phosphocholine and 1-palmitoyl-2-oleoyl- sn -glycero-3-phospho- l -serine at a 2:1:1 (w/w/w) ratio was resuspended by sonication in buffer containing 200 mM NaCl, 20 mM HEPES (pH 7.2 with NaOH) and 2 mM MgCl 2 . Lipids were mixed with GDN to a final detergent concentration of 2% (w/v), and lipid concentration of 20 mg ml −1 for 1 h at 25 °C covered by argon gas. Purified CFTR was mixed with the lipid mixture at a protein-to-lipid ratio of 1:100 or 1:250 (w/w) and incubated at 4 °C for 2 h covered by argon gas. Methylated beta-cyclodextrin was added to the reaction at a 1.2× molar ratio to GDN. After an additional 4 h, an equivalent amount of methylated beta-cyclodextrin was added. This procedure was repeated for a total of four additions. Proteoliposomes were collected by centrifugation at 150,000 g for 45 min at 4 °C, resuspended in buffer containing 200 mM NaCl, 20 mM HEPES (pH 7.2 with NaOH) and 2 mM MgCl 2 , aliquoted, snap-frozen in liquid nitrogen and stored at −80 °C.
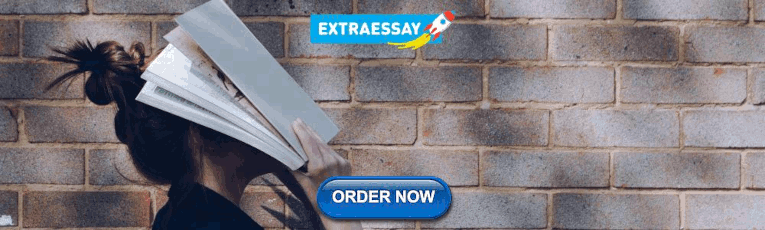
Planar lipid bilayer recording
Synthetic planar lipid bilayers were made by painting a 1,2-dioleoyl- sn -glycero-3-phosphoetanolamine, 1-palmitoyl-2-oleyl- sn -glycero-3-phosphocholine and 1-palmitoyl-2-oleoyl- sn -glycero-3-phospho- l -serine 2:1:1 (w/w/w) lipid mixture solubilized in decane across an approximately 100-µm-diameter hole on a plastic transparency. CFTR-containing proteoliposomes were phosphorylated with PKA (NEB) for 1 h at 25 °C, and then fused with the synthetic bilayers. Currents were recorded at 25 °C in symmetric buffer containing 150 mM NaCl, 2 mM MgCl 2 and 20 mM HEPES (pH 7.2 with NaOH), supplemented with ATP as indicated. Unless otherwise indicated voltage was clamped at 150 mV with an Axopatch 200B amplifier (Molecular Devices). Currents were low-pass filtered at 1 kHz, digitized at 20 kHz with a Digidata 1440A digitizer and recorded using the pCLAMP software suite (Molecular Devices). All displayed recordings were further low-pass filtered at 100 Hz. Data were analysed with Clampfit, GraphPad Prism and OriginPro.
Single-molecule fluorescence imaging
Imaging was carried out as outlined in ref. 50 . PEG- and biotin–PEG-passivated microfluidic chambers were incubated for 5 min with 0.8 µM streptavidin (Invitrogen) in buffer containing 0.06% (w/v) digitonin, 150 mM NaCl, 2 mM MgCl 2 and 20 mM HEPES (pH 7.2 with NaOH). CFTR was either dephosphorylated by Lambda protein phosphatase (λ, NEB) or phosphorylated by PKA (Sigma-Aldrich) before immobilization. Fluorophore-conjugated and biotin–tris-NTA-Ni 2+ -bound CFTR at 200 pM concentration was immobilized within the microfluidic chambers for 1 min, and unbound CFTR was cleared from the channel by washing with buffer. Imaging was carried out in deoxygenated imaging buffer containing 0.06% (w/v) digitonin, 150 mM NaCl, 2 mM MgCl 2 , 20 mM HEPES (pH 7.2 with NaOH), 2 mM protocatechuic acid and 50 nM protocatechuate-3,4-dioxygenase to minimize photobleaching 51 . MgCl 2 was omitted where indicated. Microfluidic chambers were reused several times in the same day by dissociating the immobilized protein with 300 mM imidazole. Experiments were carried out at 25 °C.
For imaging of proteoliposome-reconstituted CFTR, vesicles containing fluorophore-labelled CFTR were extruded through 400-nm and then 100-nm polycarbonate filters (Whatman). The vesicles were then incubated with 1 µM biotin–tris-NTA-Ni 2+ . Excess biotin–tris-NTA-Ni 2+ was removed by pelleting the vesicles by ultracentrifugation at 150,000 g for 45 min, removing the supernatant and resuspending in buffer containing 150 mM NaCl, 2 mM MgCl 2 and 20 mM HEPES (pH 7.2 with NaOH). The procedure was repeated twice. Vesicles were immobilized within the microfluidic chambers for 5 min, and unbound vesicles were cleared from the channel by washing with buffer. Imaging was carried out in deoxygenated imaging buffer containing 150 mM NaCl, 2 mM MgCl 2 , 20 mM HEPES (pH 7.2 with NaOH), 2 mM protocatechuic acid and 50 nM protocatechuate-3,4-dioxygenase.
Single-molecule imaging was carried out using a custom-built wide-field, prism-based total internal reflection fluorescence microscope. LD555 fluorophores were excited with an evanescent wave generated using a 532-nm laser (Opus, Laser Quantum). Emitted fluorescence from LD555 and LD655 was collected with a 1.27 NA 60× water-immersion objective (Nikon), spectrally separated using a T635lpxr dichroic (Chroma), and imaged onto two Fusion sCMOS cameras (Hamamatsu) with integration periods of 10 or 100 ms.
Single-molecule FRET data analysis
Single-molecule fluorescence data were analysed using SPARTAN analysis software in MATLAB 21 . FRET trajectories were calculated from the emitted donor and acceptor fluorescence intensities ( I D and I A , respectively) as E FRET = I A /( I A + I D ). FRET trajectories were selected for further analysis on the basis of the following criteria: single-step donor photobleaching; a signal-to-noise ratio >8; fewer than 4 donor-blinking events; and FRET efficiency above baseline for at least 50 frames. Further, single-molecule traces exhibiting FRET values above 0.8 were excluded from analysis. This subpopulation was insensitive to phosphorylation and nucleotide, and probably reflected denatured molecules. For kinetic analysis, traces were also manually curated to remove obvious photophysical artefacts. FRET trajectories were idealized using the segmental k- means algorithm 52 with a model containing two non-zero-FRET states with FRET values of 0.25 ± 0.1 and 0.48 ± 0.1. Data were further analysed with GraphPad Prism and OriginPro.
Electron microscopy data acquisition and processing
Dephosphorylated wild-type CFTR directly from gel filtration was concentrated to 5.5 mg ml −1 . Concentrations of 3 mM ATP and 3 mM fluorinated Fos-choline-8 were added to the sample immediately before application onto Quantifoil R1.2/1.3 400 mesh Au grids and then vitrification using a Vitrobot Mark IV (FEI).
Cryo-EM images were collected with a 300-keV Titan Krios transmission electron microscope equipped with a Gatan K2 Summit detector using SerialEM 53 . A total of 3,501 micrographs were collected in superresolution mode with a nominal defocus range of 0.8–2.5 µm. Micrographs had a physical pixel size of 1.03 Å (0.515 Å superresolution pixel size). Micrographs were recorded with 10-s exposure (0.2 s per frame) with a dose rate of 8 electrons per pixel per second.
Image stacks were gain-normalized, binned by 2, and corrected for beam-induced specimen motion with MotionCor2 (ref. 54 ). Contrast transfer function estimation was carried out using GCTF 55 . Images with estimated resolutions below 4.5 Å were removed. Particles were initially picked with the Laplacian-of-Gaussian implementation in RELION 56 . Selected two-dimensional classes from this particle set were then used for template-based particle picking. The 710,322 picked particles were cleaned by several rounds of two- and three-dimensional classification. A total of 157,629 particles were included in the final refined map.
Reporting summary
Further information on research design is available in the Nature Portfolio Reporting Summary linked to this article.
Data availability
The cryo-EM map has been deposited in the Electron Microscopy Data Bank under the accession code EMD-29637 . The corresponding atomic model has been deposited in the Protein Data Bank under accession code 8FZQ . The data that support the findings of this study are available from the authors upon reasonable request.
Change history
04 may 2023.
A Correction to this paper has been published: https://doi.org/10.1038/s41586-023-06115-3
Cutting, G. Cystic fibrosis genetics: from molecular understanding to clinical application. Nat. Rev. Genet. 16 , 45–56 (2015).
Article CAS PubMed Google Scholar
Riordan, J. R. et al. Identification of the cystic fibrosis gene: cloning and characterization of complementary DNA. Science 245 , 1066–1073 (1989).
Article ADS CAS PubMed Google Scholar
Rommens, J. M. et al. Identification of the cystic fibrosis gene: chromosome walking and jumping. Science 245 , 1059–1065 (1989).
Gadsby, D., Vergani, P. & Csanády, L. The ABC protein turned chloride channel whose failure causes cystic fibrosis. Nature 440 , 477–483 (2006).
Article ADS CAS PubMed PubMed Central Google Scholar
Csanády, L., Vergani, P. & Gadsby, D. C. Structure, gating, and regulation of the CFTR anion channel. Physiol. Rev. 99 , 707–738 (2019).
Article PubMed Google Scholar
Hwang, T. C. et al. Structural mechanisms of CFTR function and dysfunction. J. Gen. Physiol. 150 , 539–570 (2018).
Article CAS PubMed PubMed Central Google Scholar
Cheng, S. H. et al. Phosphorylation of the R domain by cAMP-dependent protein kinase regulates the CFTR chloride channel. Cell 66 , 1027–1036 (1991).
Carson, M. R., Travis, S. M. & Welsh, M. J. The two nucleotide-binding domains of cystic fibrosis transmembrane conductance regulator (CFTR) have distinct functions in controlling channel activity. J. Biol. Chem. 270 , 1711–1717 (1995).
Aleksandrov, L., Aleksandrov, A. A., Chang, X. B. & Riordan, J. R. The first nucleotide binding domain of cystic fibrosis transmembrane conductance regulator is a site of stable nucleotide interaction, whereas the second is a site of rapid turnover. J. Biol. Chem. 277 , 15419–15425 (2002).
Csanády, L., Vergani, P. & Gadsby, D. C. Strict coupling between CFTR’s catalytic cycle and gating of its Cl- ion pore revealed by distributions of open channel burst durations. Proc. Natl Acad. Sci. USA 107 , 1241–1246 (2010).
Article ADS PubMed Google Scholar
Szollosi, A., Vergani, P. & Csanády, L. Involvement of F1296 and N1303 of CFTR in induced-fit conformational change in response to ATP binding at NBD2. J. Gen. Physiol. 136 , 407–423 (2010).
Wang, W. et al. ATP-independent CFTR channel gating and allosteric modulation by phosphorylation. Proc. Natl Acad. Sci. USA 107 , 3888–3893 (2010).
Liu, F., Zhang, Z., Csanády, L., Gadsby, D. C. & Chen, J. Molecular structure of the human CFTR ion channel. Cell 169 , 85–95 (2017).
Zhang, Z., Liu, F. & Chen, J. Molecular structure of the ATP-bound, phosphorylated human CFTR. Proc. Natl Acad. Sci. USA 115 , 12757–12762 (2018).
Vergani, P., Lockless, S. W., Nairn, A. C. & Gadsby, D. C. CFTR channel opening by ATP-driven tight dimerization of its nucleotide-binding domains. Nature 433 , 876–880 (2005).
Vergani, P., Nairn, A. C. & Gadsby, D. C. On the mechanism of MgATP-dependent gating of CFTR Cl - channels. J. Gen. Physiol. 121 , 17–36 (2003).
Chaves, L. A. & Gadsby, D. C. Cysteine accessibility probes timing and extent of NBD separation along the dimer interface in gating CFTR channels. J. Gen. Physiol. 145 , 261–283 (2015).
Tsai, M. F., Li, M. & Hwang, T. C. Stable ATP binding mediated by a partial NBD dimer of the CFTR chloride channel. J. Gen. Physiol. 135 , 399–414 (2010).
Jih, K. Y. & Hwang, T. C. Nonequilibrium gating of CFTR on an equilibrium theme. Physiology 27 , 351–361 (2012).
Pati, A. K. et al. Tuning the Baird aromatic triplet-state energy of cyclooctatetraene to maximize the self-healing mechanism in organic fluorophores. Proc. Natl Acad. Sci. USA 117 , 24305–24315 (2020).
Juette, M. F. et al. Single-molecule imaging of non-equilibrium molecular ensembles on the millisecond timescale. Nat. Methods 13 , 341–344 (2016).
Article PubMed PubMed Central Google Scholar
Winter, M. C., Sheppard, D. N., Carson, M. R. & Welsh, M. J. Effect of ATP concentration on CFTR Cl - channels: a kinetic analysis of channel regulation. Biophys. J. 66 , 1398–1403 (1994).
Anderson, M. P. & Welsh, M. J. Regulation by ATP and ADP of CFTR chloride channels that contain mutant nucleotide-binding domains. Science 257 , 1701–1704 (1992).
Randak, C. O. & Welsh, M. J. ADP inhibits function of the ABC transporter cystic fibrosis transmembrane conductance regulator via its adenylate kinase activity. Proc. Natl Acad. Sci. USA 102 , 2216–2220 (2005).
Basso, C., Vergani, P., Nairn, A. C. & Gadsby, D. C. Prolonged nonhydrolytic interaction of nucleotide with CFTR’s NH 2 -terminal nucleotide binding domain and its role in channel gating. J. Gen. Physiol. 122 , 333–348 (2003).
Zhou, Z. et al. The two ATP binding sites of cystic fibrosis transmembrane conductance regulator (CFTR) play distinct roles in gating kinetics and energetics. J. Gen. Physiol. 128 , 413–422 (2006).
Baukrowitz, T., Hwang, T. C., Nairn, A. C. & Gadsby, D. C. Coupling of CFTR Cl- channel gating to an ATP hydrolysis cycle. Neuron 12 , 473–482 (1994).
Lin, W. Y., Jih, K. Y. & Hwang, T. C. A single amino acid substitution in CFTR converts ATP to an inhibitory ligand. J. Gen. Physiol. 144 , 311–320 (2014).
Yeh, H. I. et al. Functional stability of CFTR depends on tight binding of ATP at its degenerate ATP-binding site. J. Physiol. 599 , 4625–4642 (2021).
Shteinberg, M., Haq, I. J., Polineni, D. & Davies, J. C. Cystic fibrosis. Lancet 397 , 2195–2211 (2021).
Rees, D., Johnson, E. & Lewinson, O. ABC transporters: the power to change. Nat. Rev. Mol. Cell Biol. 10 , 218–227 (2009).
Bompadre, S. G., Sohma, Y., Li, M. & Hwang, T. C. G551D and G1349D, two CF-associated mutations in the signature sequences of CFTR, exhibit distinct gating defects. J. Gen. Physiol. 129 , 285–298 (2007).
Wang C. et al. Mechanism of dual pharmacological correction and potentiation of human CFTR. Preprint at bioRxiv https://doi.org/10.1101/2022.10.10.510913 (2022).
Zhang, Z., Liu, F. & Chen, J. Conformational changes of CFTR upon phosphorylation and ATP binding. Cell 170 , 483–491 (2017).
Hadida, S. et al. Discovery of N-(2,4-di-tert-butyl-5-hydroxyphenyl)-4-oxo-1,4-dihydroquinoline-3-carboxamide (VX-770, ivacaftor), a potent and orally bioavailable CFTR potentiator. J. Med. Chem. 57 , 9776–9795 (2014).
Van der Plas, S. E. et al. Discovery of N-(3-carbamoyl-5,5,7,7-tetramethyl-5,7-dihydro-4H-thieno[2,3-c]pyran-2-yl)-lH-pyrazole-5-carboxamide (GLPG1837), a novel potentiator which can open class III mutant cystic fibrosis transmembrane conductance regulator (CFTR) channels to a high extent. J. Med. Chem. 61 , 1425–1435 (2018).
Liu, F. et al. Structural identification of a hotspot on CFTR for potentiation. Science 364 , 1184–1188 (2019).
Yeh, H. I., Sohma, Y., Conrath, K. & Hwang, T. C. A common mechanism for CFTR potentiators. J. Gen. Physiol. 149 , 1105–1118 (2017).
Jih, K. Y. & Hwang, T. C. Vx-770 potentiates CFTR function by promoting decoupling between the gating cycle and ATP hydrolysis cycle. Proc. Natl Acad. Sci. USA 110 , 4404–4409 (2013).
Yu, H. et al. Ivacaftor potentiation of multiple CFTR channels with gating mutations. J. Cyst. Fibros. 11 , 237–245 (2012).
Langron, E., Prins, S. & Vergani, P. Potentiation of the cystic fibrosis transmembrane conductance regulator by VX-770 involves stabilization of the pre-hydrolytic, O1 state. Br. J. Pharmacol. 175 , 3990–4002 (2018).
Lin, W. Y., Sohma, Y. & Hwang, T. C. Synergistic potentiation of cystic fibrosis transmembrane conductance regulator gating by two chemically distinct potentiators, ivacaftor (VX-770) and 5-nitro-2-(3-phenylpropylamino) benzoate. Mol. Pharmacol. 90 , 275–285 (2016).
Gunderson, K. L. & Kopito, R. R. Conformational states of CFTR associated with channel gating: the role ATP binding and hydrolysis. Cell 82 , 231–239 (1995).
Bompadre, S. G. et al. CFTR gating II: effects of nucleotide binding on the stability of open states. J. Gen. Physiol. 125 , 377–394 (2005).
Powe, A. C. Jr, Al-Nakkash, L., Li, M. & Hwang, T. C. Mutation of Walker-A lysine 464 in cystic fibrosis transmembrane conductance regulator reveals functional interaction between its nucleotide-binding domains. J. Physiol. 539 , 333–346 (2002).
Mihályi, C., Töröcsik, B. & Csanády, L. Obligate coupling of CFTR pore opening to tight nucleotide-binding domain dimerization. eLife 5 , e18164 (2016).
Zhang, Z. & Chen, J. Atomic structure of the cystic fibrosis transmembrane conductance regulator. Cell 167 , 1586–1597 (2016).
Goehring, A. et al. Screening and large-scale expression of membrane proteins in mammalian cells for structural studies. Nat. Protoc. 9 , 2574–2585 (2014).
Scharschmidt, B. F., Keeffe, E. B., Blankenship, N. M. & Ockner, R. K. Validation of a recording spectrophotometric method for measurement of membrane-associated Mg- and NaK-ATPase activity. J. Lab. Clin. Med. 93 , 790–799 (1979).
CAS PubMed Google Scholar
Dyla, M. et al. Dynamics of P-type ATPase transport revealed by single-molecule FRET. Nature 551 , 346–351 (2017).
Aitken, C. E., Marshall, R. A. & Puglisi, J. D. An oxygen scavenging system for improvement of dye stability in single-molecule fluorescence experiments. Biophys. J. 94 , 1826–1835 (2008).
Qin, F. Restoration of single-channel currents using the segmental k-means method based on hidden Markov modeling. Biophys. J. 86 , 1488–1501 (2004).
Mastronarde, D. N. Automated electron microscope tomography using robust prediction of specimen movements. J. Struct. Biol. 152 , 36–51 (2005).
Zheng, S. Q. et al. MotionCor2: anisotropic correction of beam-induced motion for improved cryo-electron microscopy. Nat. Methods 14 , 331–332 (2017).
Zhang, K. Gctf: real-time CTF determination and correction. J. Struct. Biol. 193 , 1–12 (2016).
Zivanov, J. et al. New tools for automated high-resolution cryo-EM structure determination in RELION-3. eLife 7 , e42166 (2018).
Fitzgerald, G. A. et al. Quantifying secondary transport at single-molecule resolution. Nature 575 , 528–534 (2019).
Download references
Acknowledgements
We acknowledge the support from the Single-Molecule Imaging Center at St. Jude Children’s Research Hospital and the Evelyn Gruss Lipper Cryo-EM Resource Center of The Rockefeller University. We thank L. Csanády and I. Chen for their comments on the manuscript. This work was financially supported by HHMI (to J.C.) and the National Institutes of Health (GM079238 to S.C.B.).
Author information
Authors and affiliations.
Laboratory of Membrane Biology and Biophysics, The Rockefeller University, New York, NY, USA
Jesper Levring & Jue Chen
Department of Structural Biology, St. Jude Children’s Research Hospital, Memphis, TN, USA
Daniel S. Terry, Zeliha Kilic & Scott C. Blanchard
Department of Physiology and Biophysics, Weill Cornell Medicine, New York, NY, USA
Gabriel Fitzgerald
Howard Hughes Medical Institute, The Rockefeller University, New York, NY, USA
You can also search for this author in PubMed Google Scholar
Contributions
J.L. prepared all CFTR samples, carried out electrophysiology experiments, ATP turnover assays and cryo-EM experiments, and analysed the data. J.L. carried out the single-molecule FRET experiments and analysed the data, with assistance from D.S.T. and G.F. Z.K. carried out kinetic modelling and simulations with assistance from all other authors. All authors contributed to writing the manuscript. S.C.B. and J.C. oversaw the project.
Corresponding authors
Correspondence to Scott C. Blanchard or Jue Chen .
Ethics declarations
Competing interests.
S.C.B. has an equity interest in Lumidyne Technologies. The other authors declare no competing interests.
Peer review
Peer review information.
Nature thanks Cédric Govaerts and the other, anonymous, reviewer(s) for their contribution to the peer review of this work. Peer reviewer reports are available.
Additional information
Publisher’s note Springer Nature remains neutral with regard to jurisdictional claims in published maps and institutional affiliations.
Extended data figures and tables
Extended data fig. 1 functional characterization of cftr fret ..
a . Design of FRET variant CFTR. The positions of native substituted, native retained, and novel cysteines are indicated with black, grey, and magenta spheres, respectively. The dashed line represents the structurally unresolved part of the R-domain. The C1458S substitution in the disordered C-terminus is not annotated. b . Example recordings showing Protein Kinase A (PKA)-activated, ATP-dependent, and GLPG1837-stimulated current from C-terminally GFP-fused wild-type and FRET variant CFTR in inside-out excised patches. 3 mM ATP, 300 nM PKA, and 10 µM GLPG1837 were used. c . Relative GLPG1837-mediated stimulation of wild-type and FRET variant CFTR currents in excised inside-out patches. Whiskers represent minima and maxima and boxes represent 25 th , 50 th , and 75 th percentiles for 21 (wild-type) or 6 (FRET variant) patches. d . Single exponential time-constants of current activation for wild-type and FRET variant CFTR after application of 3 mM ATP. Data represent means and standard errors for 42 (wild-type) or 11 (FRET variant) patches. e-g . Exponential time-constants of the fast ( e ) and slow ( f ) components of current relaxation after ATP withdrawal, and the relative weight of the slow component ( g ), for wild-type and FRET variant CFTR. Data represent means and standard errors for 38 (wild-type) or 3 (FRET variant) patches. h . Steady state ATPase activity of wild-type and fluorophore-labelled FRET variant CFTR determined from bulk experiments. Data points represent means and standard errors for 10 (wild-type) or 3 (FRET variant) measurements. i . Voltage families of individual wild-type (left) and fluorophore-labelled FRET variant (right) CFTR channels reconstituted in synthetic lipid bilayers. The membrane potential (V m ) is indicated using physiological convention. j . Current-voltage relationships of wild-type and fluorophore-labelled FRET variant CFTR. Data points represent means and standard errors for 3 (wild-type) or 7-18 (FRET variant) channels. k . Example recordings of wild-type and fluorophore-labelled FRET variant CFTR reconstituted in synthetic lipid bilayers. CFTR was phosphorylated with PKA prior to fusion with the bilayer. The recording was performed with 3 mM ATP before (left) and after (right) addition of 10 µM GLPG1837. l . Open probabilities of the phosphorylated wild-type and fluorophore-labelled FRET variant CFTR in 3 mM ATP. Data points represent means and standard errors for 39 (wild-type) or 17 (FRET variant) channels. m . Open dwell time distributions for the phosphorylated wild-type and fluorophore-labelled FRET variant CFTR in 3 mM ATP. Statistical significance was tested using two-tailed Student’s t-test (ns: not significant).
Extended Data Fig. 2 Site-specific labelling and surface-immobilization of CFTR.
a . Site-specificity of fluorophore-conjugation to novel cysteines. Cysteine-less CFTR, with or without T388C/S1435C substitutions, was incubated with maleimide-conjugated LD555 and LD655 fluorophores. The products were separated by SDS-PAGE. The gel was imaged for LD555 and LD655 fluorescence and then Coomassie-stained. Labeling was >90 % specific to the two introduced cysteines. The experiment was repeated twice independently with similar results. For gel source data, see Supplementary Fig. 1 . b . Gel-filtration profiles of wild-type CFTR and fluorophore-labelled CFTR FRET . c . Schematic drawing of the immobilization strategy. d–f . Wide-field fluorescence images of CFTR FRET immobilized to a Streptavidin-free ( d ) or Streptavidin-coated ( e ) surface. ( f ) CFTR FRET immobilized to a Streptavidin-coated surface was washed off with 0.3 M imidazole. N denotes the number of immobilized molecules detected. g . Quantification of the specificity of Streptavidin- and His-tag-dependent immobilization. Data points represent the means and standard errors of six immobilizations. The representation of the immobilization surface in c was recreated from ref. 57 .
Extended Data Fig. 3 Phosphorylation- and nucleotide-dependence of CFTR dimerization.
a–e . Representative single-molecule traces showing donor (green) and acceptor (red) fluorescence intensities (top) and FRET (bottom) for dephosphorylated and nucleotide-free CFTR FRET ( a ), dephosphorylated CFTR FRET in the presence of 3 mM ATP ( b ), phosphorylated and nucleotide-free CFTR FRET ( c ), and phosphorylated CFTR FRET in the presence of 3 mM ATP ( d-e ). Panels a–d were collected with 100 ms integration time. Panel e was collected with 10 ms integration time. f . Contour plots of dose-dependent decrease in high FRET occupancy for phosphorylated CFTR FRET mediated by ADP in the presence of 3 mM ATP and increasing concentration of ADP. g-h . Contour plots showing time-dependent changes in FRET after rapid delivery ( g ) or withdrawal ( h ) of 10 mM ADP. CFTR FRET was phosphorylated prior to the experiments and 3 mM ATP was present throughout the experiments. i-j . Example single-molecule traces of changes in FRET dynamics of phosphorylated CFTR FRET upon rapid ADP delivery ( i ) or withdrawal ( j ). 3 mM ATP was present throughout the experiments. k . Representative titration of ADP-mediated competitive inhibition of ATP-dependent CFTR current in an inside-out excised patch. CFTR was phosphorylated prior to the recording. l . ADP dose-responses of CFTR-mediated current and high FRET state occupancy for phosphorylated CFTR in the presence of 3 mM ATP. Responses were fitted using the Hill equation (solid lines) with an IC 50 of 0.57 ± 0.05 mM for opening and an IC 50 of 2.3 ± 0.9 mM for high FRET occupancy. Hill coefficients were fixed to 1. Data represent means and standard errors for 7-11 patches and 3 FRET experiments. m . Contour plot showing time-dependent increase in FRET after application of 300 nM PKA (at the dashed line) to CFTR FRET in the presence of 3 mM ATP. n-o . Contour plots showing time-dependent decreases in FRET after application of 1 µM λ phosphatase (indicated as λ) to phosphorylated wild-type ( n ) and E1371Q ( o ) CFTR FRET , in the presence of 3 mM ATP. p-q . Example single-molecule traces of λ-dependent dephosphorylation of wild-type ( p ) and E1371Q ( q ) CFTR FRET . r . Quantification of the rate of high FRET depopulation after λ phosphatase application for wild-type and E1371Q CFTR FRET .
Extended Data Fig. 4 Structural characterization of dephosphorylated and ATP-bound CFTR.
a . Summary of cryo-EM workflow. b . cryo-EM map of dephosphorylated and ATP-bound wild-type CFTR at 4.3 Å resolution. c . Structural model of dephosphorylated and ATP-bound CFTR (PDB 8FZQ ) docked into the map. d . ATP densities in consensus (left) and degenerate (right) nucleotide binding sites.
Extended Data Fig. 5 Nucleotide-sensitivity of CFTR dynamics.
a . Representative ATP titration of CFTR-mediated current in an inside-out excised patch. C-terminally GFP-fused wild-type CFTR was phosphorylated prior to the recording. b . FRET distributions of phosphorylated CFTR FRET at the indicated ATP concentrations. c-d . Dwell time distributions of low FRET ( c ) and high FRET ( d ) states for phosphorylated CFTR FRET at the indicated ATP concentrations. e . Contour plots for phosphorylated wild-type and E1371Q variant CFTR FRET at the indicated ATP concentrations. f . Transition density plots for the same conditions as in e .
Extended Data Fig. 6 smFRET imaging of proteoliposome-reconstituted CFTR.
a . Schematic drawing of the immobilization strategy for proteoliposome-reconstituted CFTR FRET . CFTR FRET molecules may be reconstituted with either orientation in the membrane. b–f . Example single-molecule traces showing donor (green) and acceptor (red) fluorescence intensities (top) and FRET (bottom) for phosphorylated and proteoliposome-reconstituted CFTR FRET . Traces are before addition of ATP ( b ), upon addition of 3 mM ATP at the first dashed line ( c ), at steady state with ATP ( d ), upon ATP withdrawal at the second dashed line ( e ), and at steady state after ATP withdrawal ( f ). The representation of the immobilization surface in a was recreated from ref. 57 .
Extended Data Fig. 7 Correlating CFTR pore opening and closure with NBD conformation.
a . Example recordings of current responses to ATP application. 3 mM ATP was rapidly applied to an inside-out excised patch containing C-terminally GFP-fused wild-type CFTR (at the dashed lined). PKA-phosphorylation was performed prior to the recording. Time-courses were fitted with monoexponential functions (red lines). b . Example measurements of FRET responses to ATP application. 3 mM ATP was rapidly applied to PKA-phosphorylated CFTR FRET (at the dashed line). Time-courses were fitted with monoexponential functions (red lines). c . Representative inside-out excised patch showing the rate of solute exchange with local perfusion. CFTR was phosphorylated prior to the recording. CFTR current was then elicited by application of 3 mM ATP. The chloride Nernst potential was switched by exchanging from a chloride-containing perfusion solution (blue bar) to a sulfate-containing perfusion solution (red bar). d . Rate of current relaxation after solute exchange. Data represent means (solid black line) and standard errors (grey shaded area) for 7 patches. The time-course was fit with a monoexponential function (red line). e . Rate of relaxation in fluorescence intensity after injection (at the dashed line) of a DNA-conjugated Cy2 fluorophore into the imaging chamber. The experimental time-course (black points) was fitted with a monoexponential function (red line). f–h . Representative recordings of CFTR current relaxation upon ATP-withdrawal in inside-out excised patches: ATP withdrawal from wild-type CFTR, BeF 3 − -trapped wild-type CFTR, and E1371Q CFTR ( f ); ATP withdrawal from wild-type CFTR in the absence of Mg 2+ , and upon reapplication of Mg 2+ ( g ); ATP withdrawal from W401A CFTR ( h ). 2 mM Mg 2+ was present throughout the recordings in f and h . 3 mM ATP, 0.5 mM BeF 3 − , 2 mM Mg 2+ , and 10 mM EDTA were perfused onto the patches where indicated. CFTR was activated by application of 300 nM PKA and 3 mM ATP prior to the displayed recordings. i–k . Contour plots of FRET responses after ATP-withdrawal from phosphorylated CFTR FRET : ATP withdrawal from wild-type CFTR FRET in absence and presence of BeF 3 − or AlF 4 − and E1371Q CFTR FRET ( i ); ATP withdrawal from wild-type CFTR FRET in the absence of Mg 2+ and upon reapplication of Mg 2+ ( j ); ATP withdrawal from W401A CFTR FRET ( k ). 2 mM Mg 2+ was present throughout the experiments in i and k . 3 mM ATP, 0.5 mM BeF 3 − , 1 mM AlF 4 − , 2 mM Mg 2+ , and 10 mM EDTA were present where indicated. l . Schematic of events underlying CFTR pore closure and NBD separation. CFTR bound to two ATP molecules is dimerized, open, and competent for hydrolysis. ATP hydrolysis at the consensus site is followed by release of ADP and inorganic phosphate (P i ). The degenerate site remains occupied by ATP and this intermediate dynamically transitions between dimerized and separated conformations. The dimerized state has low open probability without ATP in the consensus site. ATP release from the degenerate site leads to stable NBD separation and channel closure. ATP is shown as blue circles.
Extended Data Fig. 8 Characterization of cystic fibrosis-associated variants.
a . Positions of cystic fibrosis-causing missense mutations. Mutated sites are shown as spheres. b–c . Representative smFRET traces of ATP delivery to and withdrawal from phosphorylated G551D ( b ) and L927P ( c ) CFTR FRET (at the vertical dashed lines). Horizontal dashed lines indicate mean FRET efficiencies of the low and high FRET states. ATP concentration was 3 mM. d . Contour plots showing time-dependent changes in FRET after application and withdrawal of 3 mM ATP (at the dashed lines) to and from phosphorylated G551D and L927P variant CFTR FRET . e . Dwell time distributions of the NBD-dimerized state for phosphorylated wild-type, L927P, and G551D CFTR FRET at 3 mM ATP. Data represent means and standard errors for 8 (wild-type) or 3 (G551D and L927P) experiments. f . FRET responses of G551D and L927P variants to ATP withdrawal (3 mM ATP to nucleotide-free at the dashed line). Relaxation to low FRET was fit with monoexponential functions (solid lines). g . Open dwell time distribution of L927P variant CFTR. The distribution was fit with a monoexponential function (orange line). The dashed line is a monoexponential fit of the open dwell time distribution of wild-type CFTR. h . Mean open dwell times for wild-type and L927P variant CFTR. Whiskers represent minima and maxima and boxes represent 25 th , 50 th , and 75 th percentiles for 13 (wild-type) or 13 (L927P) bilayer recordings. Statistical significance was tested using two-tailed Student’s t-test (**** p = 2 x 10 −8 ). i . ATP dose-responses of mean FRET for G551D and L927P variants. Responses were fitted using the Hill equation with EC 50 values of 37 ± 7 µM for G551D and 52 ± 18 µM for L927P. j-k . Contour plots of phosphorylated G551D ( j ) and L927P ( k ) CFTR FRET at the indicated ATP concentrations.
Extended Data Fig. 9 Potentiator-dependent changes in CFTR NBD dynamics.
a . Sample traces from single channel electrophysiology (black) and smFRET (blue) of wild-type, E1371Q, G551D, and L927P CFTR variants in the absence (left) or presence (right) of 10 µM GLPG1837. The bottom traces are wild-type CFTR in the absence of ATP. All other recordings were with 3 mM ATP. Measurements were performed with phosphorylated CFTR. In electrophysiology traces, upward deflections correspond to opening. b . Contour plots of phosphorylated wild-type CFTR FRET with 50 µM ATP and GLPG1837 at the indicated concentrations. c . GLPG1837 dose-response of high FRET occupancy for phosphorylated wild-type CFTR FRET at 50 µM ATP. The response was fit using the Hill equation (solid line) with an EC 50 value of 0.06 ± 0.04 µM. d . ATP dose-responses of high FRET occupancy in the presence of GLPG1837 at the indicated concentrations. The responses were fit using the Hill equation (solid lines) with EC 50 values of 55 ± 8 µM (no GLPG1837), 47 ± 8 µM (40 nM GLPG1837), 40 ± 9 µM (120 nM GLPG1837), and 30 ± 5 µM (10 µM GLPG1837). e . Rates of depopulation from the high FRET state after ATP withdrawal from phosphorylated wild-type CFTR FRET in the absence of potentiator, with 10 µM GLPG1837, or with 100 nM Ivacaftor. Data represent means and standard errors (shaded area) for 5 (without potentiator) or 3 (with GLPG1837 or Ivacaftor) experiments.
Extended Data Fig. 10 Stochastic simulation of CFTR pore and NBD dynamics.
a . Topology and rates of the simulated model. b–c . Experimental and simulated smFRET and single-channel electrophysiology traces of the wild-type ( b ) and E1371Q variant CFTR ( c ). The red dots above the simulated current traces indicate ATP hydrolysis events. d–g . Comparison of experimental and simulated dwell time distributions of pore open ( c ), pore closed ( d ), NBD dimerized ( e ), and NBD separated ( f ) states for wild-type CFTR. Experimental distributions are shown as histograms, and simulated distributions as red lines. Flicker-closures were ignored in analysis and the open and closed dwell times therefore reflect open burst and interburst dwell times, respectively. h . Experimental and simulated steady state ATP hydrolysis rates. Data represent means and standard deviations for 10 (wild-type) or 3 (E1371Q) measurements and 1500 (wild-type or E1371Q) simulated molecules.
Supplementary information
Supplementary information.
This file contains Supplementary Methods, Tables 1 and 2, Fig. 1 and References.
Reporting Summary
Supplementary video 1.
Stochastic simulation of CFTR gating. Stochastic simulation of a PKA-phosphorylated wild-type CFTR molecule at saturating ATP concentration. The topology and rates outlined in Extended Data Fig. 10a were used. Pore and NBD dynamics are indicated with simulated smFRET and single-channel electrophysiology traces. The red dots indicate ATP hydrolysis events. Step 2, rate-limiting for pore opening, is coloured blue.
Peer Review File
Rights and permissions.
Open Access This article is licensed under a Creative Commons Attribution 4.0 International License, which permits use, sharing, adaptation, distribution and reproduction in any medium or format, as long as you give appropriate credit to the original author(s) and the source, provide a link to the Creative Commons licence, and indicate if changes were made. The images or other third party material in this article are included in the article’s Creative Commons licence, unless indicated otherwise in a credit line to the material. If material is not included in the article’s Creative Commons licence and your intended use is not permitted by statutory regulation or exceeds the permitted use, you will need to obtain permission directly from the copyright holder. To view a copy of this licence, visit http://creativecommons.org/licenses/by/4.0/ .
Reprints and permissions
About this article
Cite this article.
Levring, J., Terry, D.S., Kilic, Z. et al. CFTR function, pathology and pharmacology at single-molecule resolution. Nature 616 , 606–614 (2023). https://doi.org/10.1038/s41586-023-05854-7
Download citation
Received : 29 June 2022
Accepted : 16 February 2023
Published : 22 March 2023
Issue Date : 20 April 2023
DOI : https://doi.org/10.1038/s41586-023-05854-7
Share this article
Anyone you share the following link with will be able to read this content:
Sorry, a shareable link is not currently available for this article.
Provided by the Springer Nature SharedIt content-sharing initiative
This article is cited by
Transport mechanism of human bilirubin transporter abcc2 tuned by the inter-module regulatory domain.
- Zhi-Peng Chen
- Yuxing Chen
Nature Communications (2024)
Fluorescence resonance energy transfer at the single-molecule level
- Sanghun Yeou
Nature Reviews Methods Primers (2024)
By submitting a comment you agree to abide by our Terms and Community Guidelines . If you find something abusive or that does not comply with our terms or guidelines please flag it as inappropriate.
Quick links
- Explore articles by subject
- Guide to authors
- Editorial policies
Sign up for the Nature Briefing newsletter — what matters in science, free to your inbox daily.

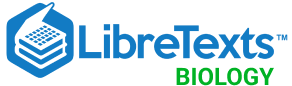
- school Campus Bookshelves
- menu_book Bookshelves
- perm_media Learning Objects
- login Login
- how_to_reg Request Instructor Account
- hub Instructor Commons
Margin Size
- Download Page (PDF)
- Download Full Book (PDF)
- Periodic Table
- Physics Constants
- Scientific Calculator
- Reference & Cite
- Tools expand_more
- Readability
selected template will load here
This action is not available.
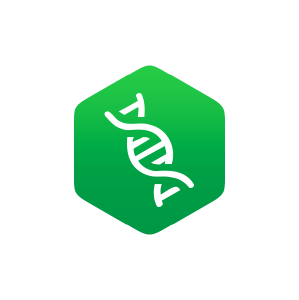
Case Study: Cystic Fibrosis - CER
- Last updated
- Save as PDF
- Page ID 26446
This page is a draft and is under active development.
\( \newcommand{\vecs}[1]{\overset { \scriptstyle \rightharpoonup} {\mathbf{#1}} } \)
\( \newcommand{\vecd}[1]{\overset{-\!-\!\rightharpoonup}{\vphantom{a}\smash {#1}}} \)
\( \newcommand{\id}{\mathrm{id}}\) \( \newcommand{\Span}{\mathrm{span}}\)
( \newcommand{\kernel}{\mathrm{null}\,}\) \( \newcommand{\range}{\mathrm{range}\,}\)
\( \newcommand{\RealPart}{\mathrm{Re}}\) \( \newcommand{\ImaginaryPart}{\mathrm{Im}}\)
\( \newcommand{\Argument}{\mathrm{Arg}}\) \( \newcommand{\norm}[1]{\| #1 \|}\)
\( \newcommand{\inner}[2]{\langle #1, #2 \rangle}\)
\( \newcommand{\Span}{\mathrm{span}}\)
\( \newcommand{\id}{\mathrm{id}}\)
\( \newcommand{\kernel}{\mathrm{null}\,}\)
\( \newcommand{\range}{\mathrm{range}\,}\)
\( \newcommand{\RealPart}{\mathrm{Re}}\)
\( \newcommand{\ImaginaryPart}{\mathrm{Im}}\)
\( \newcommand{\Argument}{\mathrm{Arg}}\)
\( \newcommand{\norm}[1]{\| #1 \|}\)
\( \newcommand{\Span}{\mathrm{span}}\) \( \newcommand{\AA}{\unicode[.8,0]{x212B}}\)
\( \newcommand{\vectorA}[1]{\vec{#1}} % arrow\)
\( \newcommand{\vectorAt}[1]{\vec{\text{#1}}} % arrow\)
\( \newcommand{\vectorB}[1]{\overset { \scriptstyle \rightharpoonup} {\mathbf{#1}} } \)
\( \newcommand{\vectorC}[1]{\textbf{#1}} \)
\( \newcommand{\vectorD}[1]{\overrightarrow{#1}} \)
\( \newcommand{\vectorDt}[1]{\overrightarrow{\text{#1}}} \)
\( \newcommand{\vectE}[1]{\overset{-\!-\!\rightharpoonup}{\vphantom{a}\smash{\mathbf {#1}}}} \)
Part I: A Case of Cystic Fibrosis
Dr. Weyland examined a six month old infant that had been admitted to University Hospital earlier in the day. The baby's parents had brought young Zoey to the emergency room because she had been suffering from a chronic cough. In addition, they said that Zoey sometimes would "wheeze" a lot more than they thought was normal for a child with a cold. Upon arriving at the emergency room, the attending pediatrician noted that salt crystals were present on Zoey's skin and called Dr. Weyland, a pediatric pulmonologist. Dr. Weyland suspects that baby Zoey may be suffering from cystic fibrosis.
CF affects more than 30,000 kids and young adults in the United States. It disrupts the normal function of epithelial cells — cells that make up the sweat glands in the skin and that also line passageways inside the lungs, pancreas, and digestive and reproductive systems.
The inherited CF gene directs the body's epithelial cells to produce a defective form of a protein called CFTR (or cystic fibrosis transmembrane conductance regulator) found in cells that line the lungs, digestive tract, sweat glands, and genitourinary system.
When the CFTR protein is defective, epithelial cells can't regulate the way that chloride ions pass across cell membranes. This disrupts the balance of salt and water needed to maintain a normal thin coating of mucus inside the lungs and other passageways. The mucus becomes thick, sticky, and hard to move, and can result in infections from bacterial colonization.
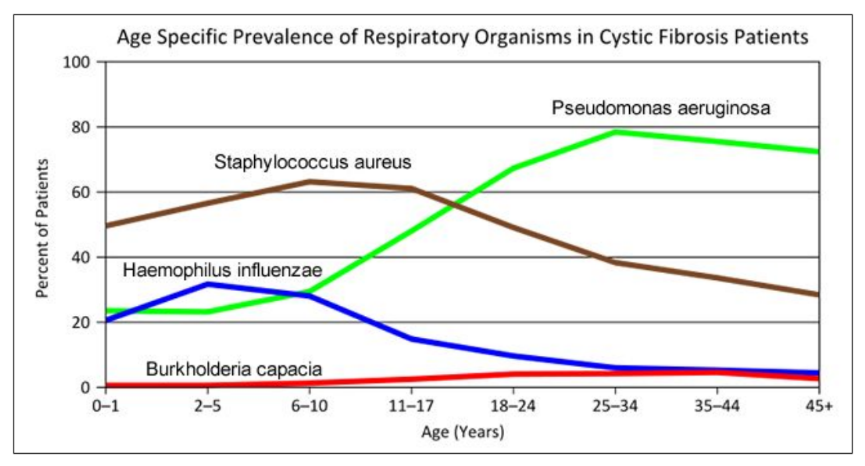
- "Woe to that child which when kissed on the forehead tastes salty. He is bewitched and soon will die" This is an old saying from the eighteenth century and describes one of the symptoms of CF (salty skin). Why do you think babies in the modern age have a better chance of survival than babies in the 18th century?
- What symptoms lead Dr. Weyland to his initial diagnosis?
- Consider the graph of infections, which organism stays relatively constant in numbers over a lifetime. What organism is most likely affecting baby Zoey?
- What do you think is the most dangerous time period for a patient with CF? Justify your answer.
Part II: CF is a disorder of the cell membrane.
Imagine a door with key and combination locks on both sides, back and front. Now imagine trying to unlock that door blind-folded. This is the challenge faced by David Gadsby, Ph.D., who for years struggled to understand the highly intricate and unusual cystic fibrosis chloride channel – a cellular doorway for salt ions that is defective in people with cystic fibrosis.
His findings, reported in a series of three recent papers in the Journal of General Physiology, detail the type and order of molecular events required to open and close the gates of the cystic fibrosis chloride channel, or as scientists call it, the cystic fibrosis transmembrane conductance regulator (CFTR).
Ultimately, the research may have medical applications, though ironically not likely for most cystic fibrosis patients. Because two-thirds of cystic fibrosis patients fail to produce the cystic fibrosis channel altogether, a cure for most is expected to result from research focused on replacing the lost channel.
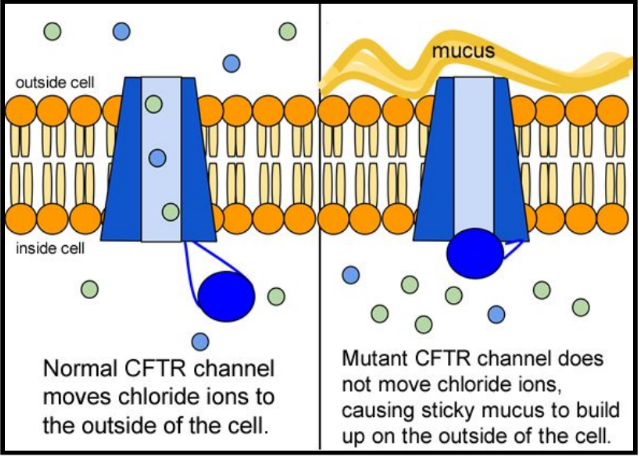
5. Suggest a molecular fix for a mutated CFTR channel. How would you correct it if you had the ability to tinker with it on a molecular level?
6. Why would treatment that targets the CFTR channel not be effective for 2⁄3 of those with cystic fibrosis?
7. Sweat glands cool the body by releasing perspiration (sweat) from the lower layers of the skin onto the surface. Sodium and chloride (salt) help carry water to the skin's surface and are then reabsorbed into the body. Why does a person with cystic fibrosis have salty tasting skin?
Part III: No cell is an island
Like people, cells need to communicate and interact with their environment to survive. One way they go about this is through pores in their outer membranes, called ion channels, which provide charged ions, such as chloride or potassium, with their own personalized cellular doorways. But, ion channels are not like open doors; instead, they are more like gateways with high-security locks that are opened and closed to carefully control the passage of their respective ions.
In the case of CFTR, chloride ions travel in and out of the cell through the channel’s guarded pore as a means to control the flow of water in and out of cells. In cystic fibrosis patients, this delicate salt/water balance is disturbed, most prominently in the lungs, resulting in thick coats of mucus that eventually spur life-threatening infections. Shown below are several mutations linked to CFTR:
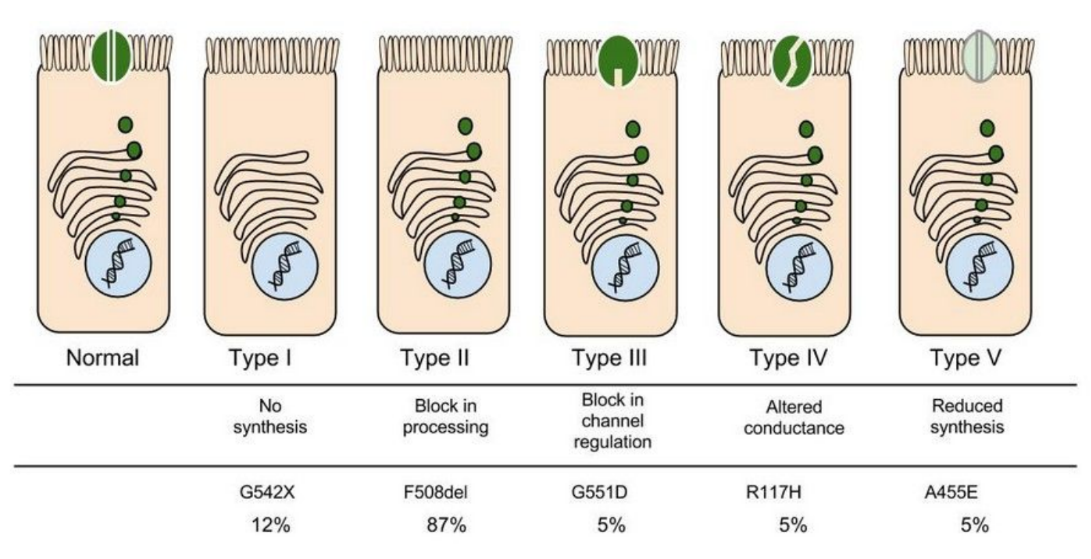
8. Which mutation do you think would be easiest to correct. Justify your answer. 9. Consider what you know about proteins, why does the “folding” of the protein matter?
Part IV: Open sesame
Among the numerous ion channels in cell membranes, there are two principal types: voltage-gated and ligand-gated. Voltage-gated channels are triggered to open and shut their doors by changes in the electric potential difference across the membrane. Ligand-gated channels, in contrast, require a special “key” to unlock their doors, which usually comes in the form of a small molecule.
CFTR is a ligand-gated channel, but it’s an unusual one. Its “key” is ATP, a small molecule that plays a critical role in the storage and release of energy within cells in the body. In addition to binding the ATP, the CFTR channel must snip a phosphate group – one of three “P’s” – off the ATP molecule to function. But when, where and how often this crucial event takes place has remains obscure.
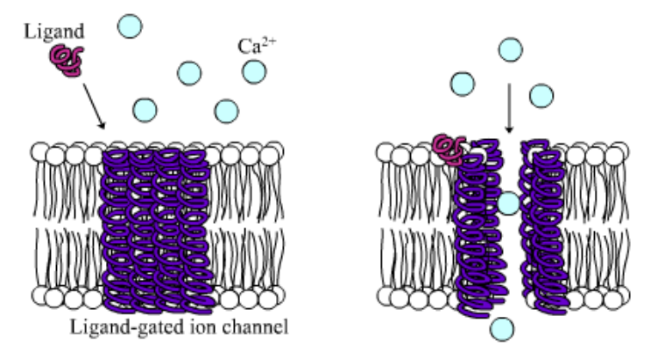
10. Compare the action of the ligand-gated channel to how an enzyme works.
11. Consider the model of the membrane channel, What could go wrong to prevent the channel from opening?
12. Where is ATP generated in the cell? How might ATP production affect the symptoms of cystic fibrosis?
13. Label the image below to show how the ligand-gated channel for CFTR works. Include a summary.
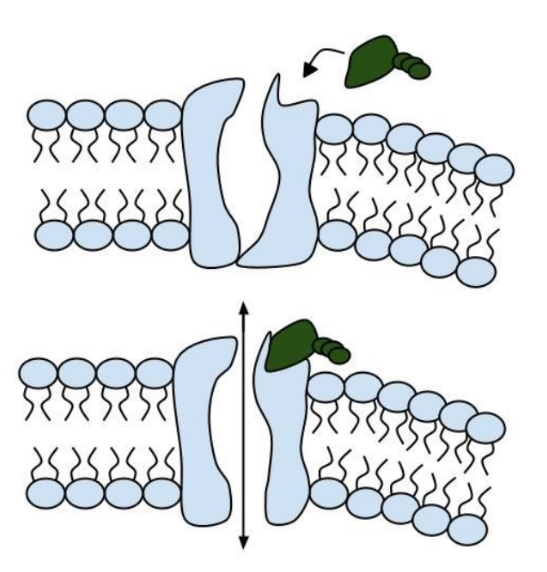
Part V: Can a Drug Treat Zoey’s Condition?
Dr. Weyland confirmed that Zoey does have cystic fibrosis and called the parents in to talk about potential treatments. “Good news, there are two experimental drugs that have shown promise in CF patients. These drugs can help Zoey clear the mucus from his lungs. Unfortunately, the drugs do not work in all cases.” The doctor gave the parents literature about the drugs and asked them to consider signing Zoey up for trials.
The Experimental Drugs
Ivacaftor TM is a potentiator that increases CFTR channel opening time. We know from the cell culture studies that this increases chloride transport by as much as 50% from baseline and restores it closer to what we would expect to observe in wild type CFTR. Basically, the drug increases CFTR activity by unlocking the gate that allows for the normal flow of salt and fluids.
In early trials, 144 patients all of whom were age over the age of 12 were treated with 150 mg of Ivacaftor twice daily. The total length of treatment was 48 weeks. Graph A shows changes in FEV (forced expiratory volume) with individuals using the drug versus a placebo. Graph B shows concentrations of chloride in patient’s sweat.
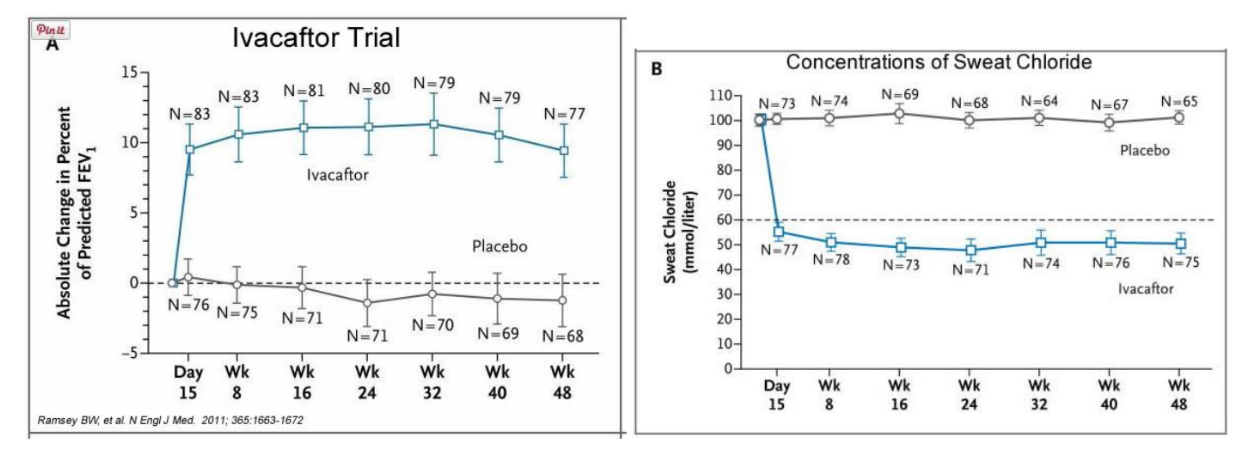
14. What is FEV? Describe a way that a doctor could take a measurement of FEV.
15. Why do you think it was important to have placebos in both of these studies?
16. Which graph do you think provides the most compelling evidence for the effectiveness of Ivacafor? Defend your choice.
17. Take a look at the mutations that can occur in the cell membrane proteins from Part III. For which mutation do you think Ivacaftor will be most effective? Justify your answer.
18. Would you sign Zoey up for clinical trials based on the evidence? What concerns would a parent have before considering an experimental drug?
Part VI: Zoey’s Mutation
Dr. Weyland calls a week later to inform the parents that genetic tests show that Zoey chromosomes show that she has two copies of the F508del mutation. This mutation, while the most common type of CF mutation, is also one that is difficult to treat with just Ivacaftor. There are still some options for treatment.
In people with the most common CF mutation, F508del, a series of problems prevents the CFTR protein from taking its correct shape and reaching its proper place on the cell surface. The cell recognizes the protein as not normal and targets it for degradation before it makes it to the cell surface. In order to treat this problem, we need to do two things: first, an agent to get the protein to the surface, and then ivacaftor (VX-770) to open up the channel and increase chloride transport. VX-809 has been identified as a way to help with the trafficking of the protein to the cell surface. When added VX-809 is added to ivacaftor (now called Lumacaftor,) the protein gets to the surface and also increases in chloride transport by increasing channel opening time.
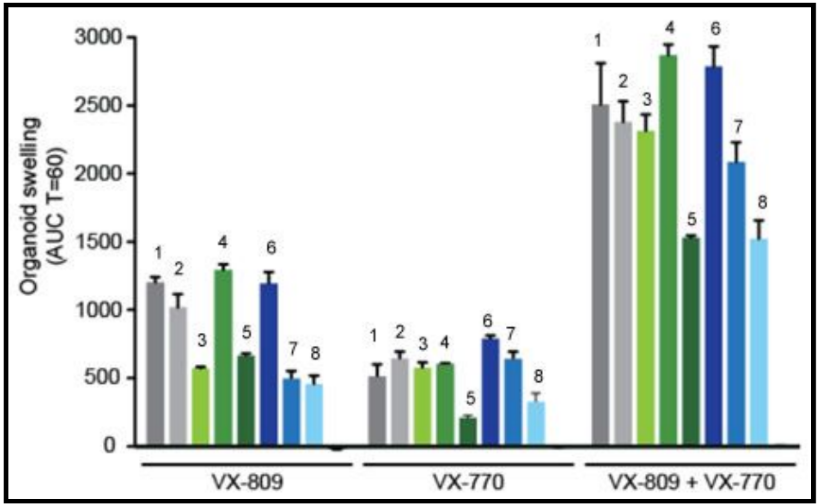
In early trials, experiments were done in-vitro, where studies were done on cell cultures to see if the drugs would affect the proteins made by the cell. General observations can be made from the cells, but drugs may not work on an individual’s phenotype. A new type of research uses ex-vivo experiments, where rectal organoids (mini-guts) were grown from rectal biopsies of the patient that would be treated with the drug. Ex-vivo experiments are personalized medicine, each person may have different correctors and potentiators evaluated using their own rectal organoids. The graph below shows how each drug works for 8 different patients (#1-#8)
19. Compare ex-vivo trials to in-vitro trials.
20. One the graph, label the group that represents Ivacaftor and Lumacaftor. What is the difference between these two drugs?
21. Complete a CER Chart. If the profile labeled #7 is Zoey, rank the possible drug treatments in order of their effectiveness for her mutation. This is your CLAIM. Provide EVIDENCE to support your claim. Provide REASONING that explains why this treatment would be more effective than other treatments and why what works for Zoey may not work for other patients. This is where you tie the graph above to everything you have learned in this case. Attach a page.

MELANIE SUE COLLINS, MD, Connecticut Children’s Medical Center, University of Connecticut School of Medicine, Hartford, Connecticut
KENIA MANSILLA-RIVERA, MD, University of Connecticut School of Medicine, Hartford, Connecticut
Am Fam Physician. 2024;109(5):388-390
Author disclosure: No relevant financial relationships.
The world of cystic fibrosis (CF) is changing. Patients are living longer, healthier lives and are now facing many of the same ongoing health challenges as people without CF. Due to significant advances in diagnostic and therapeutic options, the average age of survival for people with the condition in the United States was approximately 53 years in 2021 compared with 36 years in 2006. 1 – 3 Approximately 40,000 people in the United States are living with CF. The disease is found in patients of all racial and ethnic groups. Currently, it is most common in White people (91.2%), followed by Black (3.5%) and Asian (0.5%) people. Additionally, 10% of patients are Hispanic. 3 Lower rates of prevalence in minority groups may be due to diagnostic bias; physicians should take care to consider each CF diagnosis based on clinical presentation and not demographics.
Beginning in 2010, newborn screening for CF was universally mandated in the United States; however, there is significant variability in testing. The diagnosis of CF in patients who are racial minorities or who have mild CF or rare mutations may be missed. 4 As diagnostic effectiveness improves, many racial and ethnic minorities with CF are being identified worldwide. 5 However, these patients tend to be diagnosed later in life and have poorer outcomes. 4 , 6 , 7 Because access to therapies is key to improving quality of life and longevity, CF should be diagnosed expeditiously. 8 , 9 Regardless of the race and ethnicity of the patient, newborn screening results, or perinatal carrier testing results, it is essential to include CF in the differential diagnosis of unexplained poor weight gain, poor growth, or chronic respiratory or gastrointestinal symptoms ( Figure 1 ) .
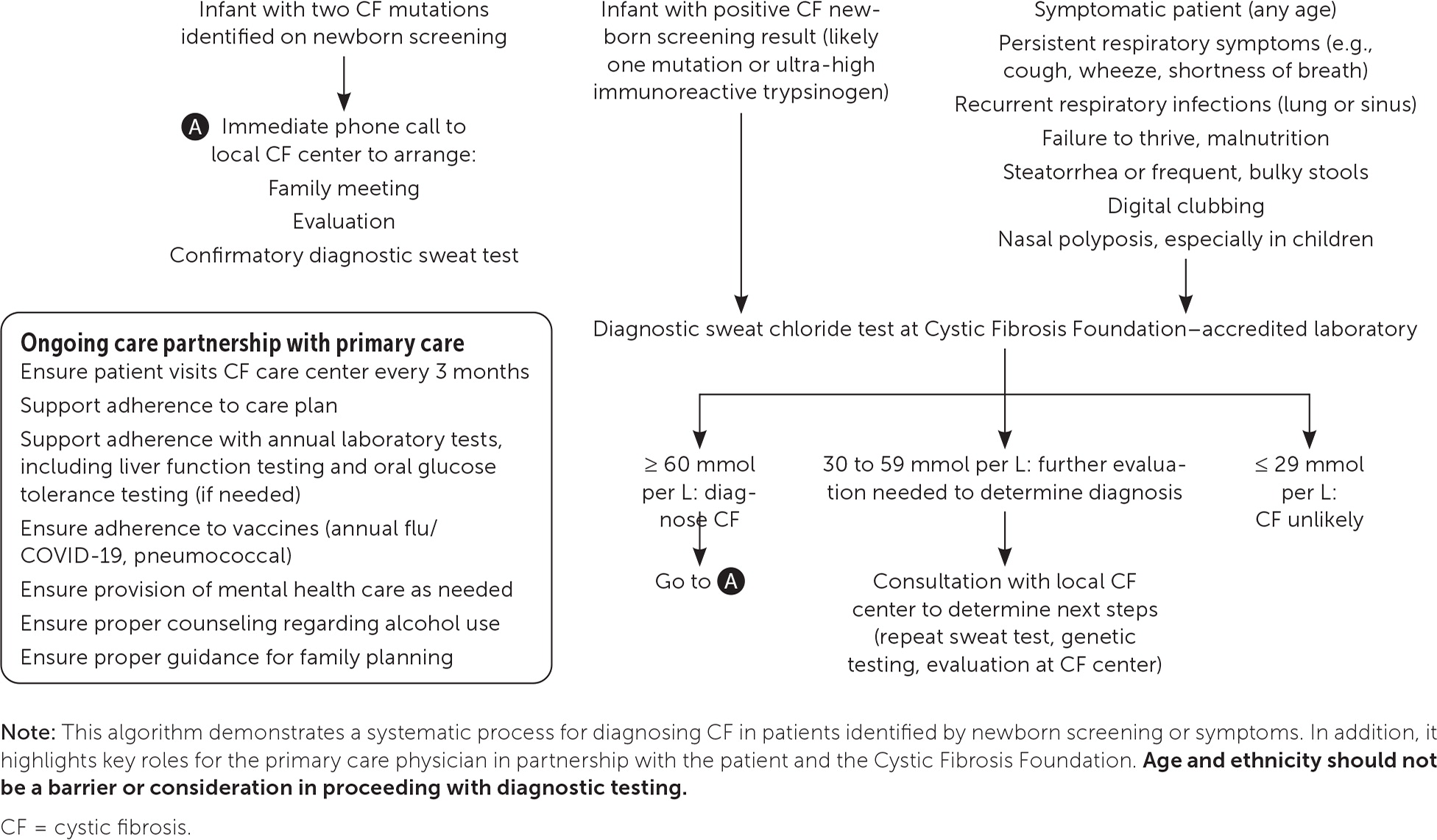
CF is an autosomal recessive disease that results in a mutation on chromosome 7. 9 This mutation is in the CF transmembrane conductance regulator protein, which is a chloride channel on the apical membrane of cells that regulates chloride flow and transport of other molecules. Mutations can be grouped into five classes based on their functional defects. 10 Because the CF transmembrane conductance regulator protein affects a variety of cell types, mutations can cause disease in multiple organ systems, most commonly the respiratory (including the upper airway), gastrointestinal, endocrine (including the pancreas), and reproductive systems. 8 There are more than 2,000 known mutations for CF with many beyond the scope of newborn screening or prenatal carrier testing programs. 11 This further supports the importance of diagnostic sweat testing in patients with symptoms, performed at a laboratory and care center accredited by the Cystic Fibrosis Foundation ( https://apps.cff.org/ccd ).
Like any chronic condition, CF requires attention to preventive care, lifestyle modifications, disease and drug complications, drug-drug interactions, and mental health needs. A list of commonly used CF medications is provided in eTable A . In addition to guideline-directed therapeutic and diagnostic care, the Cystic Fibrosis Foundation recommends that patients receive multidisciplinary care from CF care centers every 3 months. 12 Unfortunately, some patients may not be using a CF care center or be aware of new therapeutics, and some may find care center recommendations difficult to follow. 13 Family physicians can reinforce guidelines and patient adherence to these recommendations, identify and manage complications, promote routine health maintenance and vaccinations, and assist with the transition from childhood to adult CF care.
Lung disease is a major cause of morbidity and mortality, and patients with CF require a regimen of techniques and mucolytic medications for airway clearance. 14 Aerobic exercise augments airway clearance, preserves pulmonary function, increases cardiovascular fitness, and enhances quality of life. 15 , 16 Office spirometry should be repeated over time as an indicator of lung function and other complications.
Acute complications of CF include pulmonary exacerbations, which typically result in weight loss, worsening cough and lung function, and a potential increase in supplemental oxygen needs. Depending on severity, patients with such exacerbations can be treated as outpatients with oral antibiotics, but they may require inpatient admission for intravenous antibiotic therapy to treat pulmonary exacerbations. Less common acute complications include pneumothorax and hemoptysis; these require urgent evaluation and treatment at a tertiary care facility. 17
The nutritional status of patients with CF determines long-term survival and affects lung function decline. For adults, an ideal dietary plan maintains more than 90% of ideal body weight, or a body mass index of 22 to 25 kg per m 2 , and includes supplementation with fat-soluble vitamins. 16 Because 85% of patients with CF develop pancreatic insufficiency, fecal pancreatic elastase should be tested at diagnosis and then annually. Histamine H 2 blockers or proton pump inhibitors may be used to augment pancreatic enzyme effects and treat common symptoms of gastroesophageal reflux disease. Due to high caloric needs, some patients require supplemental gastrostomy tube feeding. Constipation is common and often underdiagnosed due to abnormal stooling caused by pancreatic insufficiency. Patients with CF may also have significant liver disease, and routine monitoring of the entire gastrointestinal tract is required. 18 , 19
Other chronic complications of CF include diabetes mellitus and bone disease. CF-related diabetes occurs in about 30% of adults with CF, and A1C is not a reliable method for diagnosis or determining control; therefore, ongoing glucose monitoring is recommended, with insulin as the primary treatment. 14 , 15 Additionally, up to 12% of patients develop immune-mediated joint disease. Acute episodes present with pain and joints that are red, hot, and swollen. Episodes last 7 to 10 days and may affect any joints, usually asymmetrically. Short courses of nonsteroidal anti-inflammatory drugs and corticosteroids are useful in the management of this recurrent issue. 15 eTable B summarizes ongoing health monitoring for patients with CF.
The American College of Obstetricians and Gynecologists recommends prenatal carrier testing for CF, including prenatal patients with CF who may not know their genetic mutations. 20 Patients with CF experience issues with fertility, but improvements in quality of life and lung function with CF transmembrane conductance regulator protein modulators have enabled more women with CF to become pregnant. 21 Expert guidelines emphasize optimization of prepregnancy health, genetic counseling, and partnership with a CF care center. 21 The Cystic Fibrosis Foundation also recommends that a genetic counseling component be a part of newborn screening programs to assist families with understanding results and future family planning. 22 Due to a lack of this component in many programs, telemedicine is being explored as a more widely feasible option for genetic counseling for CF. 23
Given these advancements, family physicians play a crucial role in caring for patients with CF and connecting them with care centers and the Cystic Fibrosis Foundation website to take advantage of the new treatment and support options awaiting them there. See eTable A for information on commonly used medications and eTable B for monitoring guidance.
Farrell PM, Rock MJ, Baker MW. The impact of the CFTR gene discovery on cystic fibrosis diagnosis, counseling, and preventive therapy. Genes (Basel). 2020;11(4):401.
Allen L, Allen L, Carr SB, et al. Future therapies for cystic fibrosis. Nat Commun. 2023;14(1):693.
Cystic Fibrosis Foundation. 2022 patient registry annual data report. September 2023. Accessed March 5, 2024. https://www.cff.org/media/31216/download
Rho J, Ahn C, Gao A, et al. Disparities in mortality of Hispanic patients with cystic fibrosis in the United States. A national and regional cohort study. Am J Respir Crit Care Med. 2018;198(8):1055-1063.
Guo J, Garratt A, Hill A. Worldwide rates of diagnosis and effective treatment for cystic fibrosis. J Cyst Fibros. 2022;21(3):456-462.
Montemayor K, Jain R. Cystic fibrosis: highly effective targeted therapeutics and the impact on sex and racial disparities. Med Clin North Am. 2022;106(6):1001-1012.
Hutchins K, Barr E, Bellcross C, et al. Evaluating differences in the disease experiences of minority adults with cystic fibrosis. J Patient Exp. 2022;9 : 23743735221112629.
Farrell PM, White TB, Ren CL, et al. Diagnosis of cystic fibrosis: consensus guidelines from the Cystic Fibrosis Foundation [published correction appears in J Pediatr . 2017; 184: 243]. J Pediatr. 2017;181S:S4-S15.e1.
Burgener EB, Cornfield DN. Delivering a new future for people with cystic fibrosis. Pediatrics. 2023;152(4):e2023062985.
De Boeck K, Zolin A, Cuppens H, et al. The relative frequency of CFTR mutation classes in European patients with cystic fibrosis. J Cyst Fibros. 2014;13(4):403-409.
Clinical and Functional Translation of CFTR. Updated April 7, 2023. Accessed March 5, 2024. https://cftr2.org
Cystic Fibrosis Foundation. CF care center visits. Accessed March 5, 2024. https://www.cff.org/managing-cf/cf-care-center-visits
Egan ME. Non-modulator therapies: developing a therapy for every cystic fibrosis patient. Clin Chest Med. 2022;43(4):717-725.
Kapnadak SG, Dimango E, Hadjiliadis D, et al. Cystic Fibrosis Foundation consensus guidelines for the care of individuals with advanced cystic fibrosis lung disease. J Cyst Fibros. 2020;19(3):344-354.
Chin M, Brennan AL, Bell SC. Emerging nonpulmonary complications for adults with cystic fibrosis: adult cystic fibrosis series. Chest. 2022;161(5):1211-1224.
Yankaskas JR, Marshall BC, Sufian B, et al. Cystic fibrosis adult care: consensus conference report. Chest. 2004;125(1 suppl):1S-39S.
Flume PA, Mogayzel PJ, Robinson KA, et al.; Clinical Practice Guidelines for Pulmonary Therapies Committee; Cystic Fibrosis Foundation Pulmonary Therapies Committee. Cystic fibrosis pulmonary guidelines: pulmonary complications: hemoptysis and pneumothorax. Am J Respir Crit Care Med. 2010;182(3):298-306.
Hadjiliadis D, Khoruts A, Zauber AG, et al.; Cystic Fibrosis Colorectal Cancer Screening Task Force. Cystic fibrosis colorectal cancer screening consensus recommendations. Gastroenterology. 2018;154(3):736-745.e14.
Kamal N, Surana P, Koh C. Liver disease in patients with cystic fibrosis. Curr Opin Gastroenterol. 2018;34(3):146-151.
Hopkins MK, Dugoff L, Kuller JA. Guidelines for cystic fibrosis carrier screening in the prenatal/preconception period. Obstet Gynecol Surv. 2022;77(10):606-610.
Jain R, Kazmerski TM, Zuckerwise LC, et al. Pregnancy in cystic fibrosis: review of the literature and expert recommendations. J Cyst Fibros. 2022;21(3):387-395.
Langfelder-Schwind E, Raraigh KS, Parad RB; CF Newborn Screening Genetic Counseling Workgroup. Genetic counseling access for parents of newborns who screen positive for cystic fibrosis: consensus guidelines. Pediatr Pulmonol. 2022;57(4):894-902.
Stalker HJ, Jonasson AR, Hopfer SM, et al. Improvement in cystic fibrosis newborn screening program outcomes with genetic counseling via telemedicine. Pediatr Pulmonol. 2023;58(12):3478-3486.
Continue Reading
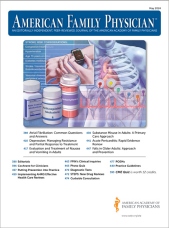
More in AFP
Copyright © 2024 by the American Academy of Family Physicians.
This content is owned by the AAFP. A person viewing it online may make one printout of the material and may use that printout only for his or her personal, non-commercial reference. This material may not otherwise be downloaded, copied, printed, stored, transmitted or reproduced in any medium, whether now known or later invented, except as authorized in writing by the AAFP. See permissions for copyright questions and/or permission requests.
Copyright © 2024 American Academy of Family Physicians. All Rights Reserved.

An official website of the United States government
The .gov means it's official. Federal government websites often end in .gov or .mil. Before sharing sensitive information, make sure you're on a federal government site.
The site is secure. The https:// ensures that you are connecting to the official website and that any information you provide is encrypted and transmitted securely.
- Publications
- Account settings
- Browse Titles
NCBI Bookshelf. A service of the National Library of Medicine, National Institutes of Health.
StatPearls [Internet]. Treasure Island (FL): StatPearls Publishing; 2024 Jan-.

StatPearls [Internet].
Cystic fibrosis.
Elaine Yu ; Sandeep Sharma .
Affiliations
Last Update: August 8, 2022 .
- Continuing Education Activity
Until relatively modern times, cystic fibrosis was poorly understood. In 1949, Lowe et al. postulated that cystic fibrosis must be caused by a genetic defect from the autosomal recessive pattern of inheritance of the disease. High levels of salt in the sweat of patients with cystic fibrosis suggested an abnormality in electrolyte transport from the sweat gland. Researchers now know that cystic fibrosis is an autosomal recessive disorder of exocrine gland function most commonly affecting persons of Northern European descent at a rate of 1:3500. It is a chronic disease that frequently leads to chronic sinopulmonary infections and pancreatic insufficiency. The most common cause of death is end-stage lung disease. This activity reviews the workup of cystic fibrosis and describes the role of health professionals working together to manage this condition.
- Review the pathophysiology of cystic fibrosis.
- Describe the presentation of cystic fibrosis.
- Summarize the treatment of cystic fibrosis.
- Outline the workup of cystic fibrosis and the role of health professionals working together to manage this condition.
- Introduction
Since ancient times, children around the world have been afflicted with cystic fibrosis that leads to shortened lifespans. In medieval Europe, these children were believed to be cursed by witches and doomed to die. The curse that became folklore pronounced, “Woe to the child who tastes salty from a kiss on the brow, for he is cursed and soon will die.” Salty skin was a sign of an impending illness without cause or cure. Until relatively modern times, cystic fibrosis was poorly understood. In 1949, Lowe et al. postulated that cystic fibrosis must be caused by a genetic defect from the autosomal recessive pattern of inheritance of the disease. High levels of salt in the sweat of patients with cystic fibrosis suggested an abnormality in electrolyte transport from the sweat gland. Quinton postulated that sweat ducts in these patients were impermeable to chloride. Further studies led to the hypothesis that the faulty chloride channel must be situated in the apical membranes of the lung surface or glandular epithelium to explain the respiratory and systemic organ failure associated with cystic fibrosis. Researchers now know that cystic fibrosis is an autosomal recessive disorder of exocrine gland function most commonly affecting persons of Northern European descent at a rate of 1 in 3500. It is a chronic disease that frequently leads to chronic sinopulmonary infections and pancreatic insufficiency. The most common cause of death is end-stage lung disease. [1] [2] [3] [4]
CF is caused by a genetic mutation in a gene on chromosome 7 that codes for a protein transmembrane conductance regulator (CFTR) protein, which functions as a transmembrane cAMP-activated chloride channel. Both copies of the gene are mutated in clinical disease.
There are over 2000 different mutations in the CFTR gene that can cause disease. These mutations are divided into five classes:
- Defective protein synthesis
- Defective protein processing
- Disordered regulation
- Defective chloride conductance
- Accelerated channel turnover [5]
- Epidemiology
The most common mutation is delta F508, which is found in 70% of American white patients with CF and two-thirds of all cases worldwide. This mutation is a class 2 mutation of abnormal folding of the CFTR protein, leading to premature destruction within the Golgi apparatus. The delta F508 mutation commonly leads to exocrine pancreatic insufficiency and a higher likelihood of meconium ileus. [6]
- Pathophysiology
Class 1 dysfunction is the result of nonsense, frameshift, or splice-site mutation, which leads to premature termination of the mRNA sequence. This fails to translate the genetic information into a protein product with a subsequent total absence of CFTR protein, and approximately 2% to 5% of cystic fibrosis cases result.
Class 2 dysfunction results in abnormal post-translational processing of the CFTR protein. This step in protein processing is essential for the proper intracellular transit of the protein. As a result, CFTR is unable to be moved to the correct cellular location.
Class 3 dysfunction is characterized by diminished protein activity in response to intracellular signaling. The result is a fully formed protein channel in the cellular membrane that is non-functional.
Class 4 dysfunction is when the protein is produced and correctly localized to the cell surface. However, the rate of chloride ion flow and the duration of channel activation after stimulation is decreased from normal.
Class 5 dysfunction is the net decreased concentration of CFTR channels in the cellular membrane as a result of rapid degradation by cellular processes. It includes mutations that alter the stability of mRNA and others that alter the stability of the mature CFTR protein.
The result of all mutations is decreased secretion of chloride and consequently increased resorption of sodium into the cellular space. The increased sodium reabsorption leads to increased water resorption and manifests as thicker mucus secretions on epithelial linings and more viscous secretions from exocrine tissues. Thickened mucus secretions in nearly every organ system involved result in mucous plugging with obstruction pathologies. The most commonly affected organs include the sinuses, lungs, pancreas, biliary and hepatic systems, intestines, and sweat glands.
Sinus disease occurs when secretion viscosity increases which obstruct the sinus ostia. Additional processes often exist here. These include ciliary dysfunction, increased inflammatory mediators, and increased bacterial colonization with pathogens such as Pseudomonas aeruginosa . The result of this syndrome is impaired sinus secretion clearance. Subsequently, chronic sinusitis occurs, and secondary structural damage may occur.
Lung disease occurs as a spectrum obstruction from thickened mucous secretion. It is important to note that the lungs of a CF patient are normal in utero, at birth, and after birth. Disease results as a cascade effect following infection and the subsequent inflammatory process. Mucus plugging in the bronchioles results in an obstructive lung disease clinical picture. As a result of obstruction, an environment optimal for bacterial growth is created within the airways. Bronchiectasis and further thick purulent sputum production occur. Part of the inflammatory reaction includes the production of the neutrophil interleukin-8 from epithelial cells, which functions as a secretagogue, increasing mucous secretion, thereby creating a positive feedback loop of mucous secretion with persistence of inflammation, infection, and structural damage. The result of this cascade is obstruction of the airways with the resulting failure of ventilation of the lung. Poorly managed pulmonary manifestations are the primary cause of death in patients with CF.
Pancreatic manifestations of CF are due in large part to obstruction of the pancreatic ductules by thickened secretions. On the passage of gastric contents into the proximal duodenum, the pancreatic exocrine glands are triggered to excrete pancreatic enzymes into the luminal space of the small intestines. However, increased viscosity of excretions and obstruction of the pancreatic ductules inhibits this process. The net pH of the secretions lessens due to decreased sodium bicarbonate composition lending to a lesser neutralization of the acidic stomach chyme. The lower pH chyme effectively degrades what pancreatic enzymes reach the intestinal lumen. As a result, intestinal chyme is not enzymatically processed in the intestines resulting in the pathognomonic greasy stools, colicky abdominal pain, and malabsorption of nutrients from foods. Specifically, fat-soluble vitamins A, D, E, and K are notably deficient.
Furthermore, autodigestion of the pancreas may occur as these enzymes target the pancreatic tissues. This results in pancreatitis. In severe, chronic cases, this can lead to endocrine pancreatic failure when the islets of Langerhans begin to be digested by trapped pancreatic enzymes. The lasting impacts of this spectrum of disease mimic type-1 diabetes mellitus.
Increased secretion viscosity does not spare the biliary and hepatic systems. The biliary ductules may be plugged with secretions. Obstructive cirrhosis and post-hepatic hyperbilirubinemia can occur. Secondary to this, esophageal varices, splenomegaly, and hypersplenism may occur as a result of increased hepatic portal vein pressures. Gallbladder disease is more likely to occur as a spectrum of this manifestation of CF, with up to 15% of those with cystic fibrosis having gallstones.
Intestinal involvement is typically seen in children with meconium ileus at birth and intestinal obstruction later in life. The cause of meconium ileus is multifactorial. Likely, it is due to increased fluid absorption as a result of the faulty CFTR channel with dehydration of the intestinal contents leading to constipation coupled with a change in luminal contents from normal secondary to pancreatic insufficiency as discussed above. Mechanical obstruction chronically leads to inflammation and eventual scarring and stricture formation. This may lead to further intestinal obstruction by fecal impaction or intussusception later in life.
Sweat glands offer an interesting contrast to all other tissues containing CFTR channels in that the flow of chloride is reversed. Normally, sweat glands move chloride from the extracellular space into the intracellular space. Thus, sodium and water are reabsorbed from the sweat gland tissues into the body. However, failure of the chloride channel to reabsorb chloride leads to a loss of sodium onto the skin surface and a subsequent fluid loss. This causes the pathognomonic salty skin seen with cystic fibrosis. In prolonged or warm environments or more severe cases, this may lead to hyponatremic dehydration.
In addition to acting as a chloride transport protein, other interactions of CFTR have been postulated. In the apical plasma membrane, CFTR is part of a multiprotein assembly where three of its amino acids, threonine, arginine, and leucine, act to root the protein to a region known as PDZ-type receptors. These PDZ regions have been observed to occur in multiple intracellular signaling proteins associated with the plasma membrane as well. This also roots CFTR closely to other transporters, ion channels, receptors, kinases, phosphatases, signaling molecules, and cytoskeletal elements. These interactions between CFTR and its binding proteins have been shown to be critically involved in regulating CFTR-mediated transepithelial ion transport in vitro and in vivo. It seems that these close associations may allow for CFTR to play an essential role in epithelial cells beyond as an ion channel. While not yet fully understood, modulation of CFTR in animal studies proved that inflammatory responses, maturational processing, non-chloride ion transport, and intracellular signaling are related to its function. These other interacting proteins are potential modifiers of the cystic fibrosis phenotype and may help explain the substantial differences in clinical severity between similar genotypic patients with CF. [7] [8] [9]
- History and Physical
Newborns with CF may present with meconium ileus, prolonged neonatal jaundice, or early lung infection. Infants and children with CF may present with failure to thrive and poor weight gain, anemia, undescended testicles in boys, recurrent sinopulmonary infections, and a distal intestinal obstructive syndrome with or without pancreatic insufficiency. The median age of diagnosis is 6 to 8 months; although, individuals may not exhibit clinical signs and symptoms until later.
Adults with CF often present with exacerbations of one or more symptoms. Lung manifestations of CF include chronic bronchitis, abnormal pulmonary function tests, bronchiectasis, atypical asthma, allergic bronchopulmonary aspergillosis, and colonization with Pseudomonas aeruginosa . Sinus manifestations of CF include chronic rhinosinusitis, chronic post-nasal drip, nasal polyposis, and panopacification of the paranasal sinuses. Pancreatic manifestations include pancreatic insufficiency, recurrent pancreatitis, and early-onset diabetes. Hepatobiliary manifestations include focal biliary cirrhosis, cholelithiasis, periportal fibrosis, liver cirrhosis, portal hypertension, and variceal bleeding. Musculoskeletal manifestations include kyphoscoliosis, osteopenia/osteoporosis, and arthropathy. Hematologic manifestations include iron-deficiency anemia or anemia of chronic disease leading to splenomegaly. Nephrogenic manifestations include nephrolithiasis, nephrocalcinosis, hyperoxaluria, and hypocitraturia. Dermatologic manifestations include “salty sweat,” digital clubbing, and cyanosis. Additional dermatologic conditions due to malabsorption include acrodermatitis enteropathica due to zinc deficiency and scaly dermatitis associated with fatty acid deficiency. Finally, males may be infertile due to absent vas deferens, while females have reduced fertility due to thickened cervical mucus.
In the United States, newborns are screened for CF as part of a standard newborn screening panel. Some cases of CF are discovered on prenatal ultrasound, which may demonstrate meconium peritonitis, bowel dilation, or absent gallbladder. Such findings often lead to prenatal CF carrier screening. [10] [11] [12] [13]
To diagnose CF, the following criteria must be met:
Suspicion for Cystic Fibrosis
- Sibling with cystic fibrosis
- Positive newborn screen
- Clinical symptoms consistent with CF in 1 or more organ systems
- Chronic sinopulmonary disease
- Gastrointestinal or nutritional abnormalities
- Salt loss syndromes
- Obstructive azoospermia
Evidence of CFTR Dysfunction
- Elevated sweat chloride 2 than 60 mEq/L on two occasions
- Two disease-causing CFTR mutations
- Abnormal nasal potential difference
The diagnostic pathway starts with a sweat chloride test. If normal but still symptomatic, a repeat sweat chloride test is indicated. If the test is abnormal, DNA testing is indicated. If one or less CFTR mutations are found, expanded DNA analysis is indicated. However, the finding of 2 CF-related mutations confirms the diagnosis of cystic fibrosis.
The test for immunoreactive trypsinogen (IRT), a pancreatic enzyme, increases sensitivity and specificity in screening newborns with meconium ileus for CF. IRT monitoring can be correlated with the severity of CF, and when it drops below detectable levels can indicate the need to start pancreatic enzyme replacement.
Additional diagnostics may be indicated depending on the presenting symptoms. A chest radiograph may help identify hyperinflation, bronchiectasis, abscesses, or atelectasis. Sinus radiography may demonstrate panopacification of the paranasal sinuses. Abdominal radiology may be helpful in neonates who present with meconium ileus. Bronchoalveolar lavage typically shows many neutrophils, and microbiology is commonly positive for Haemophilus influenza, Staphylococcus aureus, Pseudomonas aeruginosa, Burkholderia cepacia, Escherichia coli, or Klebsiella pneumoniae.
Pulmonary function testing is a major tool for evaluating and monitoring disease state and progression in CF. Spirometry is the commonly used pulmonary function test. It measures the volume of air exhaled during a forceful and complete exhalation after a maximal inhalation. The total exhaled volume, known as the forced vital capacity (FVC), the volume exhaled in the first second, known as the forced expiratory volume in one second (FEV1), and their ratio (FEV1/FVC) are the most critical variables reported. These values allow for interpretation of the status of the lung ventilation function. These values are compared to an expected normal for age, height, and gender to generate an expected normal value. The measured value is then calculated as a percent of normal where normal equals 100%. A normal or high FEV1 and/or a low FVC may indicate restrictive lung disease. A low FEV1 with a high FVC indicates obstructive lung disease with airway trapping. Cystic fibrosis can be expected to show air trapping patterns with low FEV1 values proportional to the severity of the disease. [14]
- Treatment / Management
Cystic fibrosis is a systemic illness that has broad implications for both quality and quantity of life when poorly controlled. Therefore, treatment should focus on optimizing function to avoid acute illness events. This should target maintaining lung function by aggressively controlling respiratory infection and clearing airways of mucus, optimizing nutritional status with pancreatic enzyme supplements and multivitamins, and finally, by managing any other health complications that may arise. This is best performed when using a team approach of specialists who are experienced in managing cystic fibrosis. [15] [16] [17] [18]
As stated previously, pulmonary disease is the most common cause of mortality in cystic fibrosis. As such, it is extremely vital to have a low threshold for diagnosis and intervention in pulmonary illness exacerbations. A pulmonary exacerbation is the worsening of lung function due to an infection. Often this is characterized by shortness of breath, fatigue, productive cough, and fever. Pulmonary function testing will worsen from baseline during an exacerbation. Any acute illness should prompt admission to a hospital facility familiar with cystic fibrosis management.
Pulmonary illness should be managed with two primary goals: treat the infection and improve oxygenation. P. aeruginosa typically induces infectious etiologies, and antibiotic therapy should have spectrum coverage against this pathogen. However, sputum culture should be obtained and a sensitivity profile obtained for the pathogens present. CF guidelines recommend at least one antibiotic to cover each pathogenic bacteria cultured from respiratory secretions and two antibiotics for P. aeruginosa infections. Mild exacerbations may be amenable to oral antibiotics, but more severe exacerbations require intravenous medication use. Inhaled antibiotics are not recommended whenever an intravenous alternative is possible. Ventilation and oxygenation should be supported through the use of inhaled bronchodilators, including albuterol and ipratropium bromide. Agents such as inhaled dornase alfa or inhaled hypertonic saline are prescribed to promote airway secretion clearance in conjunction with chest physiotherapy. Anti-inflammatory medicines such as glucocorticoids are also used to assist in opening airways to relieve the obstruction. The work of breathing should be optimized, utilizing nasal cannula oxygen when appropriate. Bilevel positive airway pressure (BiPAP) ventilation may be necessary to overcome airway trapping. Intubation with mechanical ventilation is an option but should be avoided whenever possible and used only when respiratory failure is imminent.
Chronic, supportive therapy for patients with CF includes regular pancreatic enzymes, fat-soluble vitamins (A, D, E, K), mucolytics, bronchodilators, antibiotics, and anti-inflammatory agents.
A new class of medications known as CFTR modulator therapies is designed to correct the dysfunction by improving production, intracellular processing, or function of the CFTR protein caused by the mutated gene. Each medication is targeted at a specific dysfunction caused by a specific gene mutation. Ivacaftor is used in the treatment of class 3 dysfunctions, where a mutation at G551D is the primary aberration. It acts by binding the defective CFTR protein at the cell surface and opening the chloride channel, thus restoring the proper function of the protein. This was the first medication to directly impact the protein channel rather than treating the effects of CF. Dosing for patients older than six years is 150 mg by mouth every 12 hours. Younger patients should receive weight-based dosing where those with less than 14 kg bodyweight receive 50 mg by mouth every 12 hours, and those with greater than 14 kg bodyweight should receive 75 mg by mouth every 12 hours. Lumacaftor is a chaperone molecule that was designed to move the defective CFTR protein from the intracellular organelles where it is processed and onto the cell surface. As such, it is effective in delta F508 homozygous mutation genotypes. Clinically, this medicine has no benefit when given alone. However, when combined as lumacaftor/ivacaftor has been shown in the 92-week PROGRESS study to have modest benefits for patients who tolerate the medicine in both pulmonary function testing and body mass index (BMI). This medicine combination was subsequently approved for use in a patient older than six years old. Dosing is two tablets containing lumacaftor 200 mg/ivacaftor 125 mg taken orally every 12 hours. Another medication combination currently in clinical trials is tezacaftor/ivacaftor. Tezacaftor, as a medicine, is very similar to lumacaftor in that it is a CFTR protein chaperone that improves the intracellular processing and trafficking of CFTR. Likewise, its clinical efficacy is only seen when placed in combination with ivacaftor. This medicine is showing a good safety profile, and preliminary EVOLVE and EXPAND trials are indicating less side effect profiles compared to lumacaftor/ivacaftor.
Despite major advancements in medical therapies for CF, the disease process continues to advance, and the lungs will ultimately fail prematurely from the disease burden without surgical intervention. Lung transplant is the treatment of choice for end-stage lung disease. The timing of the transplant is multifactorial. The International Society of Heart and Lung Transplantation published a list of conditions to be used when considering transplant referral and takes into consideration the 5-year predicted survival of less than 50%, the FEV1 that has fallen to 30% of predicted values, rapidly falling FEV1 despite optimal therapy, a 6-minute walk distance of less than 400 meters, the development of pulmonary hypertension in the absence of a hypoxemic exacerbation, clinical decline characterized by increasing frequency of exacerbations including acute respiratory failure requiring noninvasive ventilation, a pattern of poor clinical recovery from successive exacerbations, worsening nutritional status despite supplementation, pneumothorax, or life-threatening hemoptysis despite bronchial artery embolization. Virtually all lung transplants for CF will need replacement of both lungs. This is because a native, diseased lung would act as a source of infected secretions that would threaten the transplanted lung and possibly induce respiratory failure. It is important to note that transplantation is not a cure for CF, but it confers a prolongation of life and offers significant symptomatic relief.
Diet and Exercise
Individuals with CF are encouraged to consume a high-fat diet with supplemental fat-soluble vitamins to compensate for malabsorption. Additionally, patients living with CF are encouraged to consume a high-calorie diet to maintain a healthy weight and combat chronic inflammation and frequent infections that are commonly encountered. According to the Cystic Fibrosis Foundation, women should consume 2500 to 3000 calories a day, while men should consume 3000 to 3700 calories a day. Those living in hot climates or who participate in activities that cause sweating are encouraged to consume additional sodium in their diet. Oral feedings are preferred; however, if the intake does not meet metabolic demand as determined by continued decreases in BMI, enteral (tube) feedings should be considered. These are typically in the form of gastric tube feedings or jejunal tube feedings. Multiple control studies of enteral nutrition in patients with CF have shown benefit in the form of improved, or neutral lung function following exacerbations of illness that directly correlate with BMI. With that noted, however, no randomized studies of enteral nutrition have been performed in patients with CF to date. Parenteral nutrition may be considered only when oral or enteral nutrition is not meeting metabolic needs. Parenteral nutrition has been linked to increased risk for sepsis events and should be used sparingly. Regular exercise is encouraged in patients with CF to maintain and support lung function.
- Differential Diagnosis
- Bronchiolitis
- Bronchiectasis
- Celiac disease
- Nutritional considerations in failure to thrive
- Pediatric Aspergillosis
- Primary ciliary dyskinesia
Patients with CF are estimated to live until about the fourth decade of life before requiring lung transplantation. Lung transplantation confers a median survival of 8.5 years.
- Enhancing Healthcare Team Outcomes
Cystic fibrosis is a systemic illness that has broad implications for both quality and quantity of life when poorly controlled. Therefore, treatment should focus on optimizing function to avoid acute illness events. This should target maintaining lung function by aggressively controlling respiratory infection and clearing airways of mucus, optimizing nutritional status with pancreatic enzyme supplements and multivitamins, and finally, by managing any other health complications that may arise. This is best performed when using a team approach of specialists who are experienced in managing cystic fibrosis. Specialties that may be part of the team include pulmonologists, infectious disease, and gastroenterology. Nurses monitor patients and facilitate team communication. Pharmacists review all medications, check for drug-drug interactions, and educate patients and their families about the importance of compliance and potential side effects. [Level 5] Lung infections continue to have the highest mortality rates. For children who undergo a lung transplant, survival is increased for 6-8 years, but without a lung transplant, most children are dead within a few years.
- Review Questions
- Access free multiple choice questions on this topic.
- Comment on this article.
Periapical Chest Radiograph, Cystic Fibrosis Contributed by S Dulebohn, MD
Disclosure: Elaine Yu declares no relevant financial relationships with ineligible companies.
Disclosure: Sandeep Sharma declares no relevant financial relationships with ineligible companies.
This book is distributed under the terms of the Creative Commons Attribution-NonCommercial-NoDerivatives 4.0 International (CC BY-NC-ND 4.0) ( http://creativecommons.org/licenses/by-nc-nd/4.0/ ), which permits others to distribute the work, provided that the article is not altered or used commercially. You are not required to obtain permission to distribute this article, provided that you credit the author and journal.
- Cite this Page Yu E, Sharma S. Cystic Fibrosis. [Updated 2022 Aug 8]. In: StatPearls [Internet]. Treasure Island (FL): StatPearls Publishing; 2024 Jan-.
In this Page
Bulk download.
- Bulk download StatPearls data from FTP
Related information
- PMC PubMed Central citations
- PubMed Links to PubMed
Similar articles in PubMed
- [Chinese experts consensus statement: diagnosis and treatment of cystic fibrosis (2023)]. [Zhonghua Jie He He Hu Xi Za Zh...] [Chinese experts consensus statement: diagnosis and treatment of cystic fibrosis (2023)]. Chinese Experts Cystic Fibrosis Consensus Committee, Chinese Alliance for Rare Lung Diseases, Chinese Alliance for Rare Diseases, Bronchiectasis-China. Zhonghua Jie He He Hu Xi Za Zhi. 2023 Apr 12; 46(4):352-372.
- Review Cystic fibrosis: lessons from the sweat gland. [Physiology (Bethesda). 2007] Review Cystic fibrosis: lessons from the sweat gland. Quinton PM. Physiology (Bethesda). 2007 Jun; 22:212-25.
- Review [Cystic fibrosis--review]. [Laeknabladid. 2008] Review [Cystic fibrosis--review]. Jonsdottir B, Bergsteinsson H, Baldursson O. Laeknabladid. 2008 Dec; 94(12):831-7.
- Pathophysiology of cystic fibrosis with emphasis on salivary gland involvement. [J Dent Res. 1987] Pathophysiology of cystic fibrosis with emphasis on salivary gland involvement. Davis PB. J Dent Res. 1987 Feb; 66 Spec No:667-71.
- Cor pulmonale in cystic fibrosis. [Semin Respir Crit Care Med. 2003] Cor pulmonale in cystic fibrosis. Eckles M, Anderson P. Semin Respir Crit Care Med. 2003 Jun; 24(3):323-30.
Recent Activity
- Cystic Fibrosis - StatPearls Cystic Fibrosis - StatPearls
Your browsing activity is empty.
Activity recording is turned off.
Turn recording back on
Connect with NLM
National Library of Medicine 8600 Rockville Pike Bethesda, MD 20894
Web Policies FOIA HHS Vulnerability Disclosure
Help Accessibility Careers
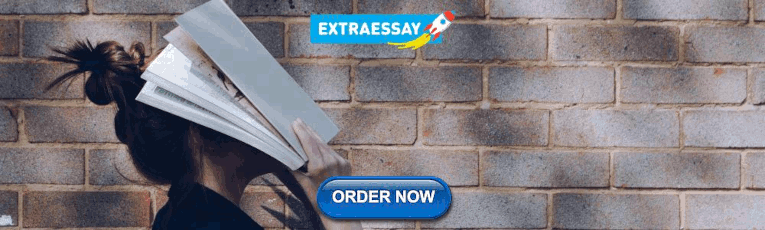
COMMENTS
Cystic fibrosis (CF) is a serious and life-shortening genetic disorder affecting approximately 70,000 persons worldwide . Respiratory failure is the foremost cause of death in CF patients, and lung transplantation is often considered in end-stage CF disease.
This case study is a follow-up to the Cystic Fibrosis Case Study where students explore how changes in transport proteins affects the movement of ions, resulting in a build-up of chloride ions and the symptoms of the disease.. Students were introduced to the idea that different mutations can cause differences in the transport proteins, but in the first version, the origin of these mutations ...
The new modulators of CFTR protein synthesis could facilitate the additional exploration needed to better understand the unfolding clinical biology of CFTR in human disease, even as they ... Crosignani A. Liver disease in cystic fibrosis: a prospective study on incidence, risk factors, and outcome. Hepatol Baltim Md. 2002; 36 (6):1374-1382 ...
In the case of F508del, the loss of a single amino acid about one-third of the way along the CFTR protein leads to two defects: the resulting protein has trouble making its way to the cell ...
The cystic fibrosis transmembrane conductance regulator (CFTR) protein helps to maintain the balance of salt and water on many surfaces in the body, such as the surface of the lung. The CFTR protein is a particular type of protein called an ion channel. In the lung, the CFTR ion channel moves chloride ions from inside the cell to outside the cell.
CFTR Gene. Cystic fibrosis transmembrane conductance regulator (CFTR) is a membrane protein and chloride channel in vertebrates that is encoded by the CFTR gene. This gene is found on chromosome 7 and is 4400 nucleotides in length. The gene encodes the CFTR protein that acts as a channel across the membrane to transport chloride ions.
A number of studies have focused specifically on proteins that interact with the cystic fibrosis protein, with the goal of restoring its normal proteostasis. Consequently, proteins involved in synthesis, folding, degradation, translocation and localization of the protein have been identified as potential therapeutic targets.
study has estimated that 0.8-13.3% of fetuses with FEB will have CF. 6 When both parents are carriers of pathogenic CFTR variants, there is a one-in-four chance (25%) of CF in the
The most prevalent mutation to the CFTR gene is the Delta-F508 mutation, accounting for approximately 70% of individuals with CF worldwide (Johns Hopkins Cystic Fibrosis Center, 2019). The Delta-F508 mutation is a deletion of only three nucleotide base pairs in the CFTR gene, resulting in the absence of the amino acid phenylalanine in the protein.
Introduction: Cystic Fibrosis (CF) is a recessive autosomal, progressive and multisystemic genetic disease. Its pathophysiological basis is the mutation in a gene whose function is to code the Cystic Fibrosis Transmembrane Conductance Regulator (CFTR) protein, responsible for regulating the transport of chlorine in the apical
This directed case study examines the molecular basis of cystic fibrosis to emphasize the relationship between the genetic code stored in a DNA sequence and the encoded protein's structure and function. Cystic fibrosis is caused by mutations in the cystic fibrosis transmembrane conductance regulator (CFTR) protein that functions to help ...
Abstract. Genetics is the branch of biology concerned with study of individual genes and how they work whereas genomics is involved with the analysis of all genes and their interactions. Both of these approaches have been applied extensively to CF. Identification of the CFTR gene initiated the dissection of CF genetics at the molecular level.
The cystic fibrosis transmembrane conductance regulator (CFTR) is an anion channel that regulates salt and fluid homeostasis across epithelial membranes 1. Alterations in CFTR cause cystic ...
Then students use this information to explain how the cell types are different. The gene therapy case study on cystic fibrosis has students model protein synthesis and challenges students to apply concepts of protein synthesis, gene expression regulation, and the effects of gene therapy on protein structure and function.
The inherited CF gene directs the body's epithelial cells to produce a defective form of a protein called CFTR (or cystic fibrosis transmembrane conductance regulator) found in cells that line the lungs, digestive tract, sweat glands, and genitourinary system. When the CFTR protein is defective, epithelial cells can't regulate the way that ...
Case Study: Cystic Fibrosis and Protein Synthesis. Request edit access.
The world of cystic fibrosis (CF) is changing. Patients are living longer, healthier lives and are now facing many of the same ongoing health challenges as people without CF. Due to significant ...
2.1. Characteristics of the human cystic fibrosis gene and encoded CFTR protein. Cystic fibrosis is caused by pathogenic mutations in a single large gene located on human chromosome 7 that encodes the cystic fibrosis transmembrane conductance regulator (CFTR) protein. 1, 2, 3 CFTR belongs to the ABC (ATP‐binding cassette) family of proteins, a large group of related proteins that share ...
the CFTR protein is a growth factor receptor four don the surface of lung cells. False; the CFTR protein is a membrane protein responsible for controlling the movement of chloride in and out of cells. in which organelle (s) does transcription occur in eukaryotes. nucleus. in prokaryotes, translation happens after transcription and RNA ...
suggest that they add extra salt to debbie's diet and watch her for dehydation. Study with Quizlet and memorize flashcards containing terms like Which statement by the mother supports the diagnosis of CF, which documentation further supports the diagnosis of CF, what information will the nurse include when teaching about the sweat test and more.
1. Introduction. Cystic fibrosis (CF) is a life-shortening and multisystem autosomal recessive disease, caused by mutation of transmembrane conductance regulator (CFTR) gene that encodes for a chloride channel expressed on the membrane of epithelial cells of the respiratory tract, intestine, hepatobiliary system, and exocrine sweat glands [1,2].The disease affects about 1 in 2500 people of ...
Name: _____ Date: ___/___/___ Period: ____ Cystic Fibrosis and Protein Synthesis Introduction Cystic fibrosis is an inherited disease that is marked by the buildup of thick, sticky mucus that can damage the lungs and many other organs. Cystic fibrosis affects the viscosity of the mucus lining of the lungs. Mucus is a collection of many substances including enzymes, proteins and muc
Since ancient times, children around the world have been afflicted with cystic fibrosis that leads to shortened lifespans. In medieval Europe, these children were believed to be cursed by witches and doomed to die. The curse that became folklore pronounced, "Woe to the child who tastes salty from a kiss on the brow, for he is cursed and soon will die." Salty skin was a sign of an impending ...