Thank you for visiting nature.com. You are using a browser version with limited support for CSS. To obtain the best experience, we recommend you use a more up to date browser (or turn off compatibility mode in Internet Explorer). In the meantime, to ensure continued support, we are displaying the site without styles and JavaScript.
- View all journals
- Explore content
- About the journal
- Publish with us
- Sign up for alerts
- Published: 17 December 2020
- Paul N. Baird 1 ,
- Seang-Mei Saw 2 , 3 , 4 ,
- Carla Lanca 2 ,
- Jeremy A. Guggenheim 5 ,
- Earl L. Smith III 6 ,
- Xiangtian Zhou 7 ,
- Kyoko-Ohno Matsui 8 ,
- Pei-Chang Wu 9 ,
- Padmaja Sankaridurg 10 ,
- Audrey Chia 11 ,
- Mohamad Rosman 12 , 13 ,
- Ecosse L. Lamoureux 13 , 14 ,
- Ryan Man 13 , 14 &
- Mingguang He 15 , 16
Nature Reviews Disease Primers volume 6 , Article number: 99 ( 2020 ) Cite this article
260 Citations
72 Altmetric
Metrics details
- Disease genetics
- Epidemiology
- Refractive errors
Myopia, also known as short-sightedness or near-sightedness, is a very common condition that typically starts in childhood. Severe forms of myopia (pathologic myopia) are associated with a risk of other associated ophthalmic problems. This disorder affects all populations and is reaching epidemic proportions in East Asia, although there are differences in prevalence between countries. Myopia is caused by both environmental and genetic risk factors. A range of myopia management and control strategies are available that can treat this condition, but it is clear that understanding the factors involved in delaying myopia onset and slowing its progression will be key to reducing the rapid rise in its global prevalence. To achieve this goal, improved data collection using wearable technology, in combination with collection and assessment of data on demographic, genetic and environmental risk factors and with artificial intelligence are needed. Improved public health strategies focusing on early detection or prevention combined with additional effective therapeutic interventions to limit myopia progression are also needed.
This is a preview of subscription content, access via your institution
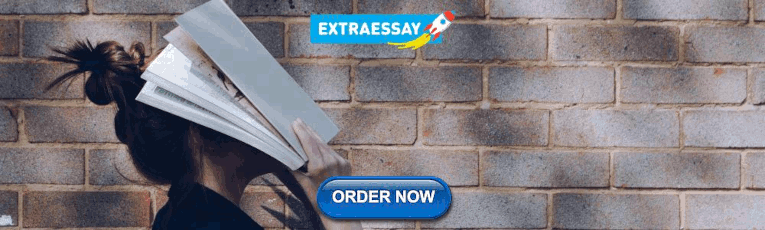
Access options
Access Nature and 54 other Nature Portfolio journals
Get Nature+, our best-value online-access subscription
24,99 € / 30 days
cancel any time
Subscribe to this journal
Receive 1 digital issues and online access to articles
92,52 € per year
only 92,52 € per issue
Buy this article
- Purchase on Springer Link
- Instant access to full article PDF
Prices may be subject to local taxes which are calculated during checkout
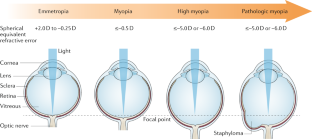
Similar content being viewed by others
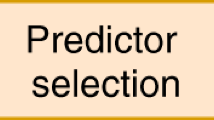
Myopia prediction: a systematic review
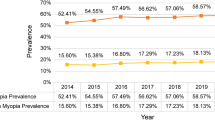
Factors associated with myopia in 19-year-old adult men in Korea between 2014 and 2020
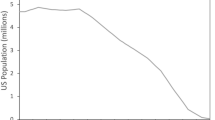
The underestimated role of myopia in uncorrectable visual impairment in the United States
Modjtahedi, B. S., Ferris, F. L., Hunter, D. G. & Fong, D. S. Public health burden and potential interventions for myopia. Ophthalmology 125 , 628–630 (2018).
PubMed Google Scholar
Morgan, I. G. et al. The epidemics of myopia: aetiology and prevention. Prog. Retin. Eye Res. 62 , 134–149 (2018). This study summarized the increasing trend of myopia prevalence and further discussed the implication and possible aetiology of the ‘epidemics’ of myopia.
Resnikoff, S. et al. Myopia - a 21st century public health issue. Invest. Ophthalmol. Vis. Sci. 60 , Mi–Mii (2019).
PubMed PubMed Central Google Scholar
Young, T. L., Metlapally, R. & Shay, A. E. Complex trait genetics of refractive error. Arch. Ophthalmol. 125 , 38–48 (2007).
CAS PubMed Google Scholar
Hashemi, H. et al. The prevalence and risk factors for keratoconus: a systematic review and meta-analysis. Cornea 39 , 263–270 (2020).
Bullimore, M. A. & Brennan, N. A. Myopia control: why each diopter matters. Optom. Vis. Sci. 96 , 463–465 (2019).
Holden, B. A. et al. Global prevalence of myopia and high myopia and temporal trends from 2000 through 2050. Ophthalmology 123 , 1036–1042 (2016). This study showed the temporal trend of myopia and high myopia prevalence supporting the notion that there will be a very high prevalence of myopia in 2050 .
Mutti, D. O., Mitchell, G. L., Moeschberger, M. L., Jones, L. A. & Zadnik, K. Parental myopia, near work, school achievement, and children’s refractive error. Invest. Ophthalmol. Vis. Sci. 43 , 3633–3640 (2002).
Huang, H.-M., Chang, D. S.-T. & Wu, P.-C. The association between near work activities and myopia in children-a systematic review and meta-analysis. PLoS ONE 10 , e0140419 (2015).
Jin, J.-X. et al. Effect of outdoor activity on myopia onset and progression in school-aged children in northeast China: the Sujiatun eye care study. BMC Ophthalmol. 15 , 73 (2015).
Saxena, R. et al. Prevalence of myopia and its risk factors in urban school children in Delhi: the North India myopia study (NIM study). PLoS ONE 10 , e0117349 (2015).
He, M. et al. Effect of time spent outdoors at school on the development of myopia among children in China: a randomized clinical trial. JAMA 314 , 1142–1148 (2015). This study is a large RCT and confirmed that outdoor activity significantly prevented myopia onset .
Wu, P. C. et al. Myopia prevention and outdoor light intensity in a school-based cluster randomized trial. Ophthalmology 125 , 1239–1250 (2018). This study was the first RCT that confirmed outdoor activity significantly prevented myopia onset and myopia progression even in moderate light intensity .
Saw, S.-M., Carkeet, A. D., Chia, K.-S., Stone, R. A. & Tan, D. T. H. Component dependent risk factors for ocular parameters in Singapore Chinese children. Ophthalmology 109 , 2065–2071 (2002).
He, M. et al. Refractive error and visual impairment in urban children in southern China. Invest. Ophthalmol. Vis. Sci. 45 , 793–799 (2004).
Yam, J. C. et al. High prevalence of myopia in children and their parents in Hong Kong Chinese population: the Hong Kong children eye study. Acta Ophthalmol. https://doi.org/10.1111/aos.14350 (2020).
Article PubMed Google Scholar
Hsu, C.-C. et al. Prevalence and risk factors for myopia in second-grade primary school children in Taipei: a population-based study. J. Chin. Med. Assoc. 79 , 625–632 (2016).
Lin, L. L. K., Shih, Y. F., Hsiao, C. K. & Chen, C. J. Prevalence of myopia in Taiwanese schoolchildren: 1983 to 2000. Ann. Acad. Med. Singap. 33 , 27–33 (2004).
Yotsukura, E. et al. Current prevalence of myopia and association of myopia with environmental factors among schoolchildren in Japan. JAMA Ophthalmol. 137 , 1233–1239 (2019).
Lim, D. H., Han, J., Chung, T., Kang, S. & Yim, H. W. Correction: the high prevalence of myopia in Korean children with influence of parental refractive errors: the 2008–2012 Korean national health and nutrition examination survey. PLoS ONE 13 , e0209876 (2018).
Anera, R. G., Soler, M., de la Cruz Cardona, J., Salas, C. & Ortiz, C. Prevalence of refractive errors in school-age children in Morocco. Clin. Exp. Ophthalmol. 37 , 191–196 (2009).
Casson, R. J. et al. Exceptionally low prevalence of refractive error and visual impairment in schoolchildren from Lao People’s Democratic Republic. Ophthalmology 119 , 2021–2027 (2012).
Dandona, R. et al. Population-based assessment of refractive error in India: the Andhra Pradesh eye disease study. Clin. Exp. Ophthalmol. 30 , 84–93 (2002).
Xiang, F. et al. Increases in the prevalence of reduced visual acuity and myopia in chinese children in Guangzhou over the past 20 years. Eye 27 , 1353–1358 (2013).
CAS PubMed PubMed Central Google Scholar
Ding, B.-Y., Shih, Y.-F., Lin, L. L. K., Hsiao, C. K. & Wang, I. J. Myopia among schoolchildren in East Asia and Singapore. Surv. Ophthalmol. 62 , 677–697 (2017).
Fan, D. S. et al. Prevalence, incidence, and progression of myopia of school children in Hong Kong. Invest. Ophthalmol. Vis. Sci. 45 , 1071–1075 (2004).
Zadnik, K. Myopia development in childhood. Optom. Vis. Sci. 74 , 603–608 (1997).
Ip, J. M. et al. Ethnic differences in refraction and ocular biometry in a population-based sample of 11–15-year-old Australian children. Eye 22 , 649–656 (2008).
Alrahili, N. H. R. et al. Prevalence of uncorrected refractive errors among children aged 3-10 years in western Saudi Arabia. Saudi Med. J. 38 , 804–810 (2017).
Carter, M. J. et al. Visual acuity and refraction by age for children of three different ethnic groups in Paraguay. Arq. Bras. Oftalmol. 76 , 94–97 (2013).
Gao, Z. et al. Refractive error in school children in an urban and rural setting in Cambodia. Ophthalmic Epidemiol. 19 , 16–22 (2012).
Murthy, G. V. S. et al. Refractive error in children in an urban population in New Delhi. Investig. Ophthalmol. Vis. Sci. 43 , 623–631 (2002).
CAS Google Scholar
Sapkota, Y. D., Adhikari, B. N., Pokharel, G. P., Poudyal, B. K. & Ellwein, L. B. The prevalence of visual impairment in school children of upper-middle socioeconomic status in Kathmandu. Ophthalmic Epidemiol. 15 , 17–23 (2008).
Jung, S. K., Lee, J. H., Kakizaki, H. & Jee, D. Prevalence of myopia and its association with body stature and educational level in 19-year-old male conscripts in Seoul, South Korea. Invest. Ophthalmol. Vis. Sci. 53 , 5579–5583 (2012).
Lee, Y. Y., Lo, C. T., Sheu, S. J. & Lin, J. L. What factors are associated with myopia in young adults? A survey study in Taiwan military conscripts. Invest. Ophthalmol. Vis. Sci. 54 , 1026–1033 (2013).
Cheng, C. Y., Hsu, W. M., Liu, J. H., Tsai, S. Y. & Chou, P. Refractive errors in an elderly chinese population in Taiwan: the Shihpai eye study. Invest. Ophthalmol. Vis. Sci. 44 , 4630–4638 (2003).
Xu, L. et al. Refractive error in urban and rural adult Chinese in Beijing. Ophthalmology 112 , 1676–1683 (2005).
Vitale, S., Ellwein, L., Cotch, M. F., Ferris, F. L. 3rd & Sperduto, R. Prevalence of refractive error in the United States, 1999–2004. Arch. Ophthalmol. 126 , 1111–1119 (2008).
Midelfart, A., Kinge, B., Midelfart, S. & Lydersen, S. Prevalence of refractive errors in young and middle-aged adults in Norway. Acta Ophthalmol. Scand. 80 , 501–505 (2002).
Bar Dayan, Y. et al. The changing prevalence of myopia in young adults: a 13-year series of population-based prevalence surveys. Invest. Ophthalmol. Vis. Sci. 46 , 2760–2765 (2005).
Williams, K. M. et al. Increasing prevalence of myopia in Europe and the impact of education. Ophthalmology 122 , 1489–1497 (2015).
Chen, M. et al. The increasing prevalence of myopia and high myopia among high school students in Fenghua city, eastern China: a 15-year population-based survey. BMC Ophthalmol. 18 , 159 (2018).
Sun, J. et al. High prevalence of myopia and high myopia in 5060 chinese university students in Shanghai. Investig. Ophthalmol. Vis. Sci. 53 , 7504–7509 (2012).
Google Scholar
Wu, J. F. et al. Refractive error, visual acuity and causes of vision loss in children in Shandong, China. The Shandong children eye study. PLoS ONE 8 , e82763 (2013).
Williams, K. M. et al. Prevalence of refractive error in Europe: the European eye epidemiology (E(3)) consortium. Eur. J. Epidemiol. 30 , 305–315 (2015).
Jacobsen, N., Jensen, H. & Goldschmidt, E. Prevalence of myopia in Danish conscripts. Acta Ophthalmol. Scand. 85 , 165–170 (2007).
French, A. N., Morgan, I. G., Burlutsky, G., Mitchell, P. & Rose, K. A. Prevalence and 5- to 6-year incidence and progression of myopia and hyperopia in Australian schoolchildren. Ophthalmology 120 , 1482–1491 (2013).
Willis, J. R. et al. The prevalence of myopic choroidal neovascularization in the United States: analysis of the IRIS(®) data registry and NHANES. Ophthalmology 123 , 1771–1782 (2016).
Asakuma, T. et al. Prevalence and risk factors for myopic retinopathy in a Japanese population: the Hisayama study. Ophthalmology 119 , 1760–1765 (2012).
Chen, S. J. et al. Prevalence and associated risk factors of myopic maculopathy in elderly Chinese: the Shihpai eye study. Invest. Ophthalmol. Vis. Sci. 53 , 4868–4873 (2012).
Vongphanit, J., Mitchell, P. & Wang, J. J. Prevalence and progression of myopic retinopathy in an older population. Ophthalmology 109 , 704–711 (2002).
Myrowitz, E. H. Juvenile myopia progression, risk factors and interventions. Saudi J. Ophthalmol. 26 , 293–297 (2012).
Fan, D. S., Lai, C., Lau, H. H., Cheung, E. Y. & Lam, D. S. Change in vision disorders among Hong Kong preschoolers in 10 years. Clin. Exp. Ophthalmol. 39 , 398–403 (2011).
Lai, Y.-H., Hsu, H.-T., Wang, H.-Z., Chang, S.-J. & Wu, W.-C. The visual status of children ages 3 to 6 years in the vision screening program in Taiwan. J. AAPOS 13 , 58–62 (2009).
Choudhury, F. et al. Prevalence and characteristics of myopic degeneration in an adult Chinese American population: the Chinese American eye study. Am. J. Ophthalmol. 187 , 34–42 (2018).
Iwase, A. et al. Prevalence and causes of low vision and blindness in a Japanese adult population: the Tajimi study. Ophthalmology 113 , 1354–1362 (2006).
Naidoo, K. S. et al. Potential lost productivity resulting from the global burden of myopia: systematic review, meta-analysis, and modeling. Ophthalmology 126 , 338–346 (2019).
Seet, B. et al. Myopia in Singapore: taking a public health approach. Br. J. Ophthalmol. 85 , 521–526 (2001).
Wong, Y.-L. et al. Prevalence, risk factors, and impact of myopic macular degeneration on visual impairment and functioning among adults in Singapore. Investig. Ophthalmol. Vis. Sci. 59 , 4603–4613 (2018).
Liu, H. H. et al. Prevalence and progression of myopic retinopathy in Chinese adults: the Beijing eye study. Ophthalmology 117 , 1763–1768 (2010).
Gao, L. Q. et al. Prevalence and characteristics of myopic retinopathy in a rural Chinese adult population: the Handan eye study. Arch. Ophthalmol. 129 , 1199–1204 (2011).
Ohno-Matsui, K. et al. International photographic classification and grading system for myopic maculopathy. Am. J. Ophthalmol. 159 , 877–883.e7 (2015).
Bez, D. et al. Association between type of educational system and prevalence and severity of myopia among male adolescents in Israel. JAMA Ophthalmol. 137 , 1–7 (2019).
PubMed Central Google Scholar
Morgan, R. W., Speakman, J. S. & Grimshaw, S. E. Inuit myopia: an environmentally induced “epidemic”? Can. Med. Assoc. J. 112 , 575–577 (1975).
He, M., Zheng, Y. & Xiang, F. Prevalence of myopia in urban and rural children in mainland China. Optom. Vis. Sci. 86 , 40–44 (2009).
Wong, T. Y., Foster, P. J., Johnson, G. J. & Seah, S. K. Education, socioeconomic status, and ocular dimensions in Chinese adults: the Tanjong Pagar Survey. Br. J. Ophthalmol. 86 , 963–968 (2002).
de Jong, P. T. V. M. Myopia: its historical contexts. Br. J. Ophthalmol. 102 , 1021–1027 (2018).
Mountjoy, E. et al. Education and myopia: assessing the direction of causality by mendelian randomisation. BMJ 361 , k2022 (2018).
Rose, K. A. et al. Outdoor activity reduces the prevalence of myopia in children. Ophthalmology 115 , 1279–1285 (2008).
Guggenheim, J. A. et al. Time outdoors and physical activity as predictors of incident myopia in childhood: a prospective cohort study. Invest. Ophthalmol. Vis. Sci. 53 , 2856–2865 (2012).
Guo, Y. et al. Outdoor activity and myopia among primary students in rural and urban regions of Beijing. Ophthalmology 120 , 277–283 (2013).
Jones-Jordan, L. A. et al. Time outdoors, visual activity, and myopia progression in juvenile-onset myopes. Invest. Ophthalmol. Vis. Sci. 53 , 7169–7175 (2012).
Wu, P. C. et al. Increased time outdoors is followed by reversal of the long-term trend to reduced visual acuity in Taiwan primary school students. Ophthalmology 127 , 1462–1469 (2020). This was the first large, long-term real-world population study that showed that outdoor policy reduced the prevalence of reduced visual acuity .
Fulk, G. W., Cyert, L. A. & Parker, D. A. Seasonal variation in myopia progression and ocular elongation. Optom. Vis. Sci. 79 , 46–51 (2002).
Ashby, R. S. & Schaeffel, F. The effect of bright light on lens compensation in chicks. Invest. Ophthalmol. Vis. Sci. 51 , 5247–5253 (2010).
Feldkaemper, M. & Schaeffel, F. An updated view on the role of dopamine in myopia. Exp. Eye Res. 114 , 106–119 (2013).
Guggenheim, J. A. et al. Does vitamin D mediate the protective effects of time outdoors on myopia? Findings from a prospective birth cohort. Invest. Ophthalmol. Vis. Sci. 55 , 8550–8558 (2014).
Jacobsen, N., Jensen, H. & Goldschmidt, E. Does the level of physical activity in university students influence development and progression of myopia? – a 2-year prospective cohort study. Invest. Ophthalmol. Vis. Sci. 49 , 1322–1327 (2008).
Torii, H. et al. Violet light exposure can be a preventive strategy against myopia progression. EBioMedicine 15 , 210–219 (2017).
Moderiano, D. et al. Influence of the time of day on axial length and choroidal thickness changes to hyperopic and myopic defocus in human eyes. Exp. Eye Res. 182 , 125–136 (2019).
Wang, Y. et al. Exposure to sunlight reduces the risk of myopia in rhesus monkeys. PLoS ONE 10 , e0127863 (2015).
Smith, E. L. III, Kee, C.-S., Ramamirtham, R., Qiao-Grider, Y. & Hung, L.-F. Peripheral vision can influence eye growth and refractive development in infant monkeys. Investig. Ophthalmol. Vis. Sci. 46 , 3965–3972 (2005). The first in a series of studies that demonstrated that, contrary to popular belief, visual signals from the fovea (that is, central retina) were not essential for the regulation of ocular growth and that signals from the peripheral retina, probably as a consequence of areal summation, can dominate refractive development .
Sorsby, A. & Fraser, G. R. Statistical note on the components of ocular refraction in twins. J. Med. Genet. 1 , 47–49 (1964). The first approach to quantify the coefficient of correlation of different ocular measures in identical and non-identical twins and that suggested that a number of genes with additive effect were inherited in myopia .
Chen, C. Y. et al. Heritability and shared environment estimates for myopia and associated ocular biometric traits: the genes in myopia (GEM) family study. Hum. Genet. 121 , 511–520 (2007).
Hammond, C. J., Snieder, H., Gilbert, C. E. & Spector, T. D. Genes and environment in refractive error: the twin eye study. Invest. Ophthalmol. Vis. Sci. 42 , 1232–1236 (2001).
Lyhne, N., Sjolie, A. K., Kyvik, K. O. & Green, A. The importance of genes and environment for ocular refraction and its determiners: a population based study among 20–45 year old twins. Br. J. Ophthalmol. 85 , 1470–1476 (2001).
Dirani, M. et al. Heritability of refractive error and ocular biometrics: the genes in myopia (GEM) twin study. Invest. Ophthalmol. Vis. Sci. 47 , 4756–4761 (2006).
Baird, P. N., Schache, M. & Dirani, M. The genes in myopia (GEM) study in understanding the aetiology of refractive errors. Prog. Retin. Eye Res. 29 , 520–542 (2010).
Tedja, M. S. et al. IMI - myopia genetics report. Invest. Ophthalmol. Vis. Sci. 60 , M89–M105 (2019).
Verhoeven, V. J. et al. Genome-wide meta-analyses of multiancestry cohorts identify multiple new susceptibility loci for refractive error and myopia. Nat. Genet. 45 , 314–318 (2013). The first large international GWAS conducted by the CREAM, based on over 37,000 individuals from 32 European and Asian studies, identified 16 new genetic loci, of which some were shared across ethnicities .
Kiefer, A. K. et al. Genome-wide analysis points to roles for extracellular matrix remodeling, the visual cycle, and neuronal development in myopia. PLoS Genet. 9 , e1003299 (2013).
Wojciechowski, R. & Hysi, P. G. Focusing in on the complex genetics of myopia. PLoS Genet. 9 , e1003442 (2013).
Tedja, M. S. et al. Genome-wide association meta-analysis highlights light-induced signaling as a driver for refractive error. Nat. Genet. 50 , 834–848 (2018).
Hysi, P. G. et al. Meta-analysis of 542,934 subjects of European ancestry identifies new genes and mechanisms predisposing to refractive error and myopia. Nat. Genet. 52 , 401–407 (2020). The largest GWAS study to date in refractive error and myopia identifying 336 novel genetic loci .
Guggenheim, J. A. et al. Assumption-free estimation of the genetic contribution to refractive error across childhood. Mol. Vis. 21 , 621–632 (2015).
Ghorbani Mojarrad, N. et al. Association between polygenic risk score and risk of myopia. JAMA Ophthalmol. 138 , 7–13 (2019).
Tideman, J. W. L. et al. When do myopia genes have their effect? Comparison of genetic risks between children and adults. Genet. Epidemiol. 40 , 756–766 (2016). This study demonstrated that different genes can impact on myopia at different ages .
Cheng, C. Y. et al. Nine loci for ocular axial length identified through genome-wide association studies, including shared loci with refractive error. Am. J. Hum. Genet. 93 , 264–277 (2013). This study identified a number of genetic loci for axial length, including some that were shared with refractive error, using a large study cohort of the CREAM .
Hysi, P. G., Wojciechowski, R., Rahi, J. S. & Hammond, C. J. Genome-wide association studies of refractive error and myopia, lessons learned, and implications for the future. Investig. Ophthalmol. Vis. Sci. 55 , 3344–3351 (2014).
Fan, Q. et al. Childhood gene-environment interactions and age-dependent effects of genetic variants associated with refractive error and myopia: the CREAM consortium. Sci. Rep. 6 , 25853 (2016).
Chen, C.-J., Cohen, B. H. & Diamond, E. L. Genetic and environmental effects on the development of myopia in Chinese twin children. Ophthalmic Paediatr. Genet. 6 , 113–119 (1985).
Saw, S.-M., Hong, C.-Y., Chia, K.-S., Stone, R. A. & Tan, D. Nearwork and myopia in young children. Lancet 357 , 390 (2001).
Dudbridge, F. & Fletcher, O. Gene-environment dependence creates spurious gene-environment interaction. Am. J. Hum. Genet. 95 , 301–307 (2014). An account of the conditions under which gene–environment dependence can arise, using as an example the potential interaction between a CYP3A variant and age at menarche on the risk of breast cancer .
Fan, Q. et al. Education influences the association between genetic variants and refractive error: a meta-analysis of five Singapore studies. Hum. Mol. Genet. 23 , 546–554 (2014). A pioneering study reporting interactions between variants in SHISA6 , GJD2 and ZMAT4 and level of education in determining susceptibility to myopia .
Wojciechowski, R., Yee, S. S., Simpson, C. L., Bailey-Wilson, J. E. & Stambolian, D. Matrix metalloproteinases and educational attainment in refractive error: evidence of gene–environment interactions in the age-related eye disease study. Ophthalmology 120 , 298–305 (2012).
Tkatchenko, A. V. et al. APLP2 regulates refractive error and myopia development in mice and humans. PLoS Genet. 11 , e1005432 (2015).
Chen, Y. P. et al. Selective breeding for susceptibility to myopia reveals a gene-environment interaction. Investig. Ophthalmol. Vis. Sci. 52 , 4003–4011 (2011).
Pozarickij, A. et al. Quantile regression analysis reveals widespread evidence for gene-environment or gene-gene interactions in myopia development. Commun. Biol. 2 , 167 (2019). A study suggesting that 88% of known GWAS loci for refractive error exhibit evidence of variance heterogeneity, which is a characteristic feature of involvement in a G× E interaction .
Huang, Y. et al. A genome-wide association study for susceptibility to visual experience-induced myopia. Invest. Ophthalmol. Vis. Sci. 60 , 559–569 (2019).
Mutti, D. O. et al. Axial growth and changes in lenticular and corneal power during emmetropization in infants. Invest. Ophthalmol. Vis. Sci. 46 , 3074–3080 (2005).
Ehrlich, D. L. et al. Infant emmetropization: longitudinal changes in refraction components from nine to twenty months of age. Optom. Vis. Sci. 74 , 822–843 (1997).
Inagaki, Y. The rapid change of corneal curvature in the neonatal period and infancy. Arch. Ophthalmol. 104 , 1026–1027 (1986).
Gordon, R. A. & Donzis, P. B. Refractive development of the human eye. Arch. Ophthalmol. 103 , 785–789 (1985).
Fledelius, H. C. & Christensen, A. C. Reappraisal of the human ocular growth curve in fetal life, infancy, and early childhood. Br. J. Ophthalmol. 80 , 918–921 (1996).
Saw, S. M. et al. Incidence and progression of myopia in Singaporean school children. Invest. Ophthalmol. Vis. Sci. 46 , 51–57 (2005).
Mutti, D. O. et al. Corneal and crystalline lens dimensions before and after myopia onset. Optom. Vis. Sci. 89 , 251–262 (2012).
Han, X., Guo, X., Lee, P. Y., Morgan, I. G. & He, M. Six-year changes in refraction and related ocular biometric factors in an adult Chinese population. PLoS ONE 12 , e0183364 (2017).
Troilo, D. et al. IMI - report on experimental models of emmetropization and myopia. Invest. Ophthalmol. Vis. Sci. 60 , M31–M88 (2019). This is the most recent and most comprehensive summary to date of the results obtained from studies of laboratory animals on the role of vision in the regulation of refractive development .
Schaeffel, F., Glasser, A. & Howland, H. C. Accommodation, refractive error and eye growth in chickens. Vis. Res. 28 , 639–657 (1988).
Wallman, J. & Winawer, J. Homeostasis of eye growth and the question of myopia. Neuron 43 , 447–468 (2004). This is the first comprehensive review of animal research on refractive development; it provides a unique perspective on how vision regulates ocular growth and refractive development .
Schaeffel, F. & Feldkaemper, M. P. Animal models in myopia research. Clin. Exp. Optom. 98 , 507–517 (2015).
Arumugam, B., Hung, L.-F., To, C.-H., Holden, B. A. & Smith, E. L. 3rd The effects of simultaneous dual focus lenses on refractive development in infant monkeys. Investig. Ophthalmol. Vis. Sci. 55 , 7423–7432 (2014).
Zhu, X. Temporal integration of visual signals in lens compensation (a review). Exp. Eye Res. 114 , 69–76 (2013).
Tse, D. Y. & To, C.-H. Graded competing regional myopic and hyperopic defocus produce summated emmetropization set points in chicks. Investig. Ophthalmol. Vis. Sci. 52 , 8056–8062 (2011).
McBrien, N. A. & Adams, D. W. A longitudinal investigation of adult-onset and adult-progression of myopia in an occupational group. Refractive and biometric findings. Invest. Ophthalmol. Vis. Sci. 38 , 321–333 (1997).
Wallman, J., Gottlieb, M. D., Rajaram, V. & Fugate-Wentzek, L. Local retinal regions control local eye growth and myopia. Science 237 , 73–77 (1987). This investigation demonstrated that ocular growth and refractive development are regulated by independent, local retinal mechanisms that operate in a regionally selective fashion, one of the most interesting and critical operational properties of the mechanisms that regulate refractive development .
Tkatchenko, T. V., Troilo, D., Benavente-Perez, A. & Tkatchenko, A. V. Gene expression in response to optical defocus of opposite signs reveals bidirectional mechanism of visually guided eye growth. PLoS Biol. 16 , e2006021 (2018).
Fischer, A. J., McGuire, J. J., Schaeffel, F. & Stell, W. K. Light- and focus-dependent expression of the transcription factor ZENK in the chick retina. Nat. Neurosci. 2 , 706–712 (1999).
Rucker, F. J. & Wallman, J. Cone signals for spectacle-lens compensation: differential responses to short and long wavelengths. Vis. Res. 48 , 1980–1991 (2008).
Foulds, W. S., Barathi, V. A. & Luu, C. D. Progressive myopia or hyperopia can be induced in chicks and reversed by manipulation of the chromaticity of ambient light. Invest. Ophthalmol. Vis. Sci. 54 , 8004–8012 (2013).
Hung, L. F., Arumugam, B., She, Z., Ostrin, L. & Smith, E. L. 3rd Narrow-band, long-wavelength lighting promotes hyperopia and retards vision-induced myopia in infant rhesus monkeys. Exp. Eye Res. 176 , 147–160 (2018).
Hagen, L. A. et al. The association between L:M cone ratio, cone opsin genes and myopia susceptibility. Vis. Res. 162 , 20–28 (2019).
Crewther, D. P. & Crewther, S. G. Refractive compensation to optical defocus depends on the temporal profile of luminance modulation of the environment. Neuroreport 13 , 1029–1032 (2002).
Aleman, A. C., Wang, M. & Schaeffel, F. Reading and myopia: contrast polarity matters. Sci. Rep. 8 , 10840 (2018).
Crewther, S. G. & Crewther, D. P. Inhibition of retinal ON/OFF systems differentially affects refractive compensation to defocus. Neuroreport 14 , 1233–1237 (2003).
Chakraborty, R. et al. ON pathway mutations increase susceptibility to form-deprivation myopia. Exp. Eye Res. 137 , 79–83 (2015).
Chakraborty, R. et al. Comparison of refractive development and retinal dopamine in OFF pathway mutant and C57BL/6J wild-type mice. Mol. Vis. 20 , 1318–1327 (2014).
Wang, M., Aleman, A. C. & Schaeffel, F. Probing the potency of artificial dynamic ON or OFF stimuli to inhibit myopia development. Invest. Ophthalmol. Vis. Sci. 60 , 2599–2611 (2019).
Chen, S. et al. Bright light suppresses form-deprivation myopia development with activation of dopamine D1 receptor signaling in the ON pathway in retina. Invest. Ophthalmol. Vis. Sci. 58 , 2306–2316 (2017).
Zhang, Y. & Wildsoet, C. F. RPE and choroid mechanisms underlying ocular growth and myopia. Prog. Mol. Biol. Transl. Sci. 134 , 221–240 (2015).
Rymer, J. & Wildsoet, C. F. The role of the retinal pigment epithelium in eye growth regulation and myopia: a review. Vis. Neurosci. 22 , 251–261 (2005). It is likely that the retinal pigment epithelium plays a critical role in the signal cascade that regulates refractive development. This review provides a comprehensive summary of what is known about the pigment epithelium and vision-dependent refractive development .
Wallman, J. et al. Moving the retina: choroidal modulation of refractive state. Vis. Res. 35 , 37–50 (1995).
Mertz, J. R. & Wallman, J. Choroidal retinoic acid synthesis: a possible mediator between refractive error and compensatory eye growth. Exp. Eye Res. 70 , 519–527 (2000).
Wildsoet, C. & Wallman, J. Choroidal and scleral mechanisms of compensation for spectacle lenses in chicks. Vis. Res. 35 , 1175–1194 (1995). This comprehensive study conclusively demonstrated that choroidal thickness was regulated by visual feedback and that changes in choroidal thickness could have a significant effect on the eye’s refractive state .
Siegwart, J. T. Jr & Norton, T. T. Regulation of the mechanical properties of tree shrew sclera by the visual environment. Vis. Res. 39 , 387–407 (1999).
Rada, J. A., Nickla, D. L. & Troilo, D. Decreased proteoglycan synthesis associated with form deprivation myopia in mature primate eyes. Invest. Ophthalmol. Vis. Sci. 41 , 2050–2058 (2000).
Siegwart, J. T. Jr & Norton, T. T. Selective regulation of MMP and TIMP mRNA levels in tree shrew sclera during minus lens compensation and recovery. Invest. Ophthalmol. Vis. Sci. 46 , 3484–3492 (2005).
McBrien, N. A. & Gentle, A. Role of the sclera in the development and pathological complications of myopia. Prog. Retin. Eye Res. 22 , 307–338 (2003).
Rada, J. A., Shelton, S. & Norton, T. T. The sclera and myopia. Exp. Eye Res. 82 , 185–200 (2006). This paper reviewed the myopia-associated scleral extracellular matrix remodelling events during myopia progression in both experimental models and humans .
Wu, H. et al. Scleral hypoxia is a target for myopia control. Proc. Natl Acad. Sci. USA 115 , e7091–e7100 (2018).
Zhang, S. et al. Changes in choroidal thickness and choroidal blood perfusion in guinea pig myopia. Invest. Ophthalmol. Vis. Sci. 60 , 3074–3083 (2019). Scleral hypoxia can develop as a result of a decline in blood perfusion in the choroid. This paper documented that choroidal blood perfusion was significantly decreased in three models of guinea pig myopia .
Yang, Y. S. & Koh, J. W. Choroidal blood flow change in eyes with high myopia. Korean J. Ophthalmol. 29 , 309–314 (2015). This paper reported a significant decrease of choroidal blood perfusion in highly myopic human eyes .
Shih, Y. F. et al. Ocular pulse amplitude in myopia. J. Ocul. Pharmacol. 7 , 83–87 (1991).
Kniestedt, C. & Stamper, R. L. Visual acuity and its measurement. Ophthalmol. Clin. North Am. 16 , 155–170 (2003).
Guo, X. et al. Normative distribution of visual acuity in 3- to 6-year-old Chinese preschoolers: the Shenzhen kindergarten eye study. Invest. Ophthalmol. Vis. Sci. 56 , 1985–1992 (2015).
Anstice, N. S. & Thompson, B. The measurement of visual acuity in children: an evidence-based update. Clin. Exp. Optom. 97 , 3–11 (2014).
Morgan, I. G., Iribarren, R., Fotouhi, A. & Grzybowski, A. Cycloplegic refraction is the gold standard for epidemiological studies. Acta Ophthalmol. 93 , 581–585 (2015).
WHO. The impact of myopia and high myopia: report of the Joint World Health Organization–Brien Holden Vision Institute Global Scientific Meeting on Myopia, University of New South Wales, Sydney, Australia, 16–18 March 2015 (World Health Organization, 2017).
Negrel, A. D., Maul, E., Pokharel, G. P., Zhao, J. & Ellwein, L. B. Refractive error study in children: sampling and measurement methods for a multi-country survey. Am. J. Ophthalmol. 129 , 421–426 (2000).
Wen, G. et al. Prevalence of myopia, hyperopia, and astigmatism in non-Hispanic white and Asian children: multi-ethnic pediatric eye disease study. Ophthalmology 120 , 2109–2116 (2013).
Lee, J. H., Jee, D., Kwon, J. W. & Lee, W. K. Prevalence and risk factors for myopia in a rural Korean population. Invest. Ophthalmol. Vis. Sci. 54 , 5466–5471 (2013).
Parssinen, O. The increased prevalence of myopia in Finland. Acta Ophthalmol. 90 , 497–502 (2012).
Pan, C. W. et al. Variation in prevalence of myopia between generations of migrant indians living in Singapore. Am. J. Ophthalmol. 154 , 376–381.e1 (2012).
Santodomingo-Rubido, J., Mallen, E. A. H., Gilmartin, B. & Wolffsohn, J. S. A new non-contact optical device for ocular biometry. Br. J. Ophthalmol. 86 , 458–462 (2002).
Hussin, H. M., Spry, P. G., Majid, M. A. & Gouws, P. Reliability and validity of the partial coherence interferometry for measurement of ocular axial length in children. Eye 20 , 1021–1024 (2006).
Curtin, B. J. & Karlin, D. B. Axial length measurements and fundus changes of the myopic eye. Am. J. Ophthalmol. 71 , 42–53 (1971).
Miller, D. G. & Singerman, L. J. Natural history of choroidal neovascularization in high myopia. Curr. Opin. Ophthalmol. 12 , 222–224 (2001).
Moriyama, M. et al. Topographic analyses of shape of eyes with pathologic myopia by high-resolution three-dimensional magnetic resonance imaging. Ophthalmology 118 , 1626–1637 (2011). This study has established a novel technique — 3D MRI of the eye — to visualize the shape of the entire globe; an entire extent of posterior staphyloma becomes visible .
Guo, X. et al. Three-dimensional eye shape, myopic maculopathy, and visual acuity: the Zhongshan ophthalmic center-Brien Holden vision institute high myopia cohort study. Ophthalmology 124 , 679–687 (2017).
Ohno-Matsui, K. & Jonas, J. B. Posterior staphyloma in pathologic myopia. Prog. Retin. Eye Res. 70 , 99–109 (2019).
Ohno-Matsui, K. Proposed classification of posterior staphylomas based on analyses of eye shape by three-dimensional magnetic resonance imaging and wide-field fundus imaging. Ophthalmology 121 , 1798–1809 (2014). Through the use of new techniques, 3D MRI and ultra-wide-field OCT, a novel classification has been established for posterior staphylomas .
Morgan, I. G., He, M. & Rose, K. A. Epidemic of pathologic myopia: what can laboratory studies and epidemiology tell us? Retina 37 , 989–997 (2016).
Ohno-Matsui, K., Lai, T. Y. Y., Cheung, C. M. G. & Lai, C. C. Updates of pathologic myopia. Prog. Retin. Eye Res. 52 , 156–187 (2016).
Ohno-Matsui, K. What is the fundamental nature of pathologic myopia? Retina 37 , 1043–1048 (2016).
Yokoi, T. et al. Peripapillary diffuse chorioretinal atrophy in children as a sign of eventual pathologic myopia in adults. Ophthalmology 123 , 1783–1787 (2016).
Ohno-Matsui, K. in Pathologic Myopia (eds Spaide, R. F., Ohno-Matsui, K., & Yannuzzi, L. A.) 187–210 (Springer, 2014).
Tong, L. et al. Screening for myopia and refractive errors using LogMAR visual acuity by optometrists and a simplified visual acuity chart by nurses. Optom. Vis. Sci. 81 , 684–691 (2004).
Priya, A. et al. Vision screening by teachers in southern Indian schools: testing a new “all class teacher” model. Ophthalmic Epidemiol. 22 , 60–65 (2015).
Sharma, A. et al. Strategies to improve the accuracy of vision measurement by teachers in rural Chinese secondary schoolchildren: Xichang Pediatric Refractive Error Study (X-PRES) report no. 6. Arch. Ophthalmol. 126 , 1434–1440 (2008).
Pattison, B. & Plymat, K. Vision screening of school children: should it be continued? Contemp. Nurse 10 , 163–171 (2001).
Ma, Y. et al. Myopia screening: combining visual acuity and noncycloplegic autorefraction. Optom. Vis. Sci. 90 , 1479–1485 (2013).
Leone, J. F., Mitchell, P., Morgan, I. G., Kifley, A. & Rose, K. A. Use of visual acuity to screen for significant refractive errors in adolescents: is it reliable? Arch. Ophthalmol. 128 , 894–899 (2010).
Tong, L. et al. Sensitivity and specificity of visual acuity screening for refractive errors in school children. Optom. Vis. Sci. 79 , 650–657 (2002).
O’Donoghue, L., Rudnicka, A. R., McClelland, J. F., Logan, N. S. & Saunders, K. J. Visual acuity measures do not reliably detect childhood refractive error – an epidemiological study. PLoS ONE 7 , e34441 (2012).
Lai, Y. H., Tseng, H. Y., Hsu, H. T., Chang, S. J. & Wang, H. Z. Uncorrected visual acuity and noncycloplegic autorefraction predict significant refractive errors in Taiwanese preschool children. Ophthalmology 120 , 271–276 (2013).
Sherwin, J. C. et al. The association between time spent outdoors and myopia in children and adolescents: a systematic review and meta-analysis. Ophthalmology 119 , 2141–2151 (2012).
Wu, P. C., Tsai, C. L., Wu, H. L., Yang, Y. H. & Kuo, H. K. Outdoor activity during class recess reduces myopia onset and progression in school children. Ophthalmology 120 , 1080–1085 (2013).
Ngo, C. S. et al. A cluster randomised controlled trial evaluating an incentive-based outdoor physical activity programme to increase outdoor time and prevent myopia in children. Ophthalmic Physiol. Opt. 34 , 362–368 (2014).
Jan, C. et al. Prevention of myopia, China. Bull. World Health Organ. 98 , 435–437 (2020).
Chung, K., Mohidin, N. & O’Leary, D. J. Undercorrection of myopia enhances rather than inhibits myopia progression. Vis. Res. 42 , 2555–2559 (2002). Undercorrection is widely practised in myopia management but this article showed that undercorrection worsened the progression of myopia .
Li, S. Y. et al. Effect of undercorrection on myopia progression in 12-year-old children. Graefes Arch. Clin. Exp. Ophthalmol. 253 , 1363–1368 (2015).
Koomson, N. Y. et al. Relationship between reduced accommodative lag and myopia progression. Optom. Vis. Sci. 93 , 683–691 (2016).
Walline, J. J., Greiner, K. L., McVey, M. E. & Jones-Jordan, L. A. Multifocal contact lens myopia control. Optom. Vis. Sci. 90 , 1207–1214 (2013).
Cheng, D., Woo, G. C., Drobe, B. & Schmid, K. L. Effect of bifocal and prismatic bifocal spectacles on myopia progression in children: three-year results of a randomized clinical trial. JAMA Ophthalmol. 132 , 258–264 (2014).
Cho, P. & Cheung, S. W. Retardation of myopia in Orthokeratology (ROMIO) study: a 2-year randomized clinical trial. Invest. Ophthalmol. Vis. Sci. 53 , 7077–7085 (2012).
Edwards, M. H., Li, R. W.-H., Lam, C. S.-Y., Lew, J. K.-F. & Yu, B. S.-Y. The Hong Kong progressive lens myopia control study: study design and main findings. Invest. Ophthalmol. Vis. Sci. 43 , 2852–2858 (2002).
Gwiazda, J. et al. A randomized clinical trial of progressive addition lenses versus single vision lenses on the progression of myopia in children. Invest. Ophthalmol. Vis. Sci. 44 , 1492–1500 (2003).
Leung, J. T. & Brown, B. Progression of myopia in Hong Kong chinese schoolchildren is slowed by wearing progressive lenses. Optom. Vis. Sci. 76 , 346–354 (1999).
Sankaridurg, P. et al. Spectacle lenses designed to reduce progression of myopia: 12-month results. Optom. Vis. Sci. 87 , 631–641 (2010).
Anstice, N. S. & Phillips, J. R. Effect of dual-focus soft contact lens wear on axial myopia progression in children. Ophthalmology 118 , 1152–1161 (2011). The study demonstrated the feasibility of slowing myopia with multifocal-type soft contact lenses .
Lam, C. S. Y., Tang, W. C., Tse, D. Y.-Y., Tang, Y. Y. & To, C. H. Defocus incorporated soft contact (DISC) lens slows myopia progression in Hong Kong Chinese schoolchildren: a 2-year randomised clinical trial. Br. J. Ophthalmol. 98 , 40–45 (2014).
Walline, J. J., Jones, L. A. & Sinnott, L. T. Corneal reshaping and myopia progression. Br. J. Ophthalmol. 93 , 1181–1185 (2009).
Cheng, X., Xu, J., Chehab, K., Exford, J. & Brennan, N. Soft contact lenses with positive spherical aberration for myopia control. Optom. Vis. Sci. 93 , 353–366 (2016).
Sankaridurg, P. et al. Myopia control with novel central and peripheral plus contact lenses and extended depth of focus contact lenses: 2 year results from a randomised clinical trial. Ophthalmic Physiol. Opt. 39 , 294–307 (2019).
Sankaridurg, P. et al. Decrease in rate of myopia progression with a contact lens designed to reduce relative peripheral hyperopia: one-year results. Invest. Ophthalmol. Vis. Sci. 52 , 9362–9367 (2011). The study demonstrated the feasibility of slowing myopia with multifocal-type soft contact lenses .
Chamberlain, P. et al. A 3-year randomized clinical trial of MiSight lenses for myopia control. Optom. Vis. Sci. 96 , 556–567 (2019).
Lam, C. S. Y. et al. Defocus incorporated multiple segments (DIMS) spectacle lenses slow myopia progression: a 2-year randomised clinical trial. Br. J. Ophthalmol. 104 , 363–368 (2020).
Kanda, H. et al. Effect of spectacle lenses designed to reduce relative peripheral hyperopia on myopia progression in Japanese children: a 2-year multicenter randomized controlled trial. Jpn. J. Ophthalmol. 62 , 537–543 (2018).
Walline, J. J. et al. Effect of high add power, medium add power, or single-vision contact lenses on myopia progression in children: the BLINK randomized clinical trial. JAMA 324 , 571–580 (2020).
Walline, J. J. et al. Contact lenses in pediatrics (CLIP) study: chair time and ocular health. Optom. Vis. Sci. 84 , 896–902 (2007).
Walline, J. J. et al. A randomized trial of the effect of soft contact lenses on myopia progression in children. Invest. Ophthalmol. Vis. Sci. 49 , 4702–4706 (2008).
Gifford, K. L. et al. IMI - clinical management guidelines report. Invest. Ophthalmol. Vis. Sci. 60 , M184–M203 (2019).
Berntsen, D. A., Sinnott, L. T., Mutti, D. O. & Zadnik, K. A randomized trial using progressive addition lenses to evaluate theories of myopia progression in children with a high lag of accommodation. Invest. Ophthalmol. Vis. Sci. 53 , 640–649 (2012).
Stapleton, F. et al. The incidence of contact lens-related microbial keratitis in Australia. Ophthalmology 115 , 1655–1662 (2008).
Ganesan, P. & Wildsoet, C. F. Pharmaceutical intervention for myopia control. Expert. Rev. Ophthalmol. 5 , 759–787 (2010).
Pineles, S. L. et al. Atropine for the prevention of myopia progression in children: a report by the American Academy of Ophthalmology. Ophthalmology 124 , 1857–1866 (2017).
Huang, J. et al. Efficacy comparison of 16 interventions for myopia control in children: a network meta-analysis. Ophthalmology 123 , 697–708 (2016). This meta-analysis compared 16 different interventions for myopia control and indicated that, while a range of interventions could significantly reduce myopia progression, the most effective interventions were pharmacological such as atropine and pirenzepine .
Li, S. M. et al. Atropine slows myopia progression more in Asian than white children by meta-analysis. Optom. Vis. Sci. 91 , 342–350 (2014). This meta-analysis of RCT results showed that atropine could significantly slow myopia progression in children with a greater effect in Asian children than in white children .
Gong, Q. et al. Efficacy and adverse effects of atropine in childhood myopia: a meta-analysis. JAMA Ophthalmol. 135 , 624–630 (2017).
Chia, A. et al. Atropine for the treatment of childhood myopia: changes after stopping atropine 0.01%, 0.1% and 0.5%. Am. J. Ophthalmol. 157 , 451–457.e1 (2014).
Chia, A., Lu, Q. S. & Tan, D. Five-year clinical trial on atropine for the treatment of myopia 2: myopia control with atropine 0.01% eyedrops. Ophthalmology 123 , 391–399 (2016).
Fang, P. C., Chung, M. Y., Yu, H. J. & Wu, P. C. Prevention of myopia onset with 0.025% atropine in premyopic children. J. Ocul. Pharmacol. Ther. 26 , 341–345 (2010).
Tong, L. et al. Atropine for the treatment of childhood myopia: effect on myopia progression after cessation of atropine. Ophthalmology 116 , 572–579 (2009).
Yam, J. C. et al. Low-concentration atropine for myopia progression (LAMP) study: a randomized, double-blinded, placebo-controlled trial of 0.05%, 0.025%, and 0.01% atropine eye drops in myopia control. Ophthalmology 126 , 113–124 (2019). This study showed that the use of very low concentrations of atropine eye drops could reduce myopia progression along a concentration-dependent response, with 0.05% atropine being the most effective in controlling SE progression and AL elongation over a period of 1 year .
Yam, J. C. et al. Two-year clinical trial of the low-concentration atropine for myopia progression (LAMP) study: phase 2 report. Ophthalmology 127 , 910–919 (2019). Results of this 2-year randomized trial indicated that the effect of 0.05% atropine was double that observed with 0.01% atropine in slowing myopia progression .
Bedrossian, R. H. The effect of atropine on myopia. Ophthalmology 86 , 713–719 (1979).
Yi, S. et al. Therapeutic effect of atropine 1% in children with low myopia. J. AAPOS 19 , 426–429 (2015).
Chua, W. H. et al. Atropine for the treatment of childhood myopia. Ophthalmology 113 , 2285–2291 (2006).
Shih, Y. F. et al. Effects of different concentrations of atropine on controlling myopia in myopic children. J. Ocul. Pharmacol. Ther. 15 , 85–90 (1999). This study suggested that low concentrations of atropine at 0.1%, 0.25% and 0.5% showed significant effects on controlling myopia, with 0.5% atropine treatment being the most effective .
Lee, C. Y., Sun, C. C., Lin, Y. F. & Lin, K. K. Effects of topical atropine on intraocular pressure and myopia progression: a prospective comparative study. BMC Ophthalmol. 16 , 114 (2016).
Wu, T. E., Yang, C. C. & Chen, H. S. Does atropine use increase intraocular pressure in myopic children? Optom. Vis. Sci. 89 , E161–E167 (2012).
Chia, A., Li, W., Tan, D. & Luu, C. D. Full-field electroretinogram findings in children in the atropine treatment for myopia (ATOM2) study. Doc. Ophthalmol. 126 , 177–186 (2013).
Luu, C. D., Lau, A. M., Koh, A. H. & Tan, D. Multifocal electroretinogram in children on atropine treatment for myopia. Br. J. Ophthalmol. 89 , 151–153 (2005).
McBrien, N. A., Stell, W. K. & Carr, B. How does atropine exert its anti-myopia effects? Ophthalmic Physiol. Opt. 33 , 373–378 (2013).
Kinoshita, N. et al. Additive effects of orthokeratology and atropine 0.01% ophthalmic solution in slowing axial elongation in children with myopia: first year results. Jpn. J. Ophthalmol. 62 , 544–553 (2018).
Wan, L. et al. The synergistic effects of orthokeratology and atropine in slowing the progression of myopia. J. Clin. Med. 7 , 259 (2018).
CAS PubMed Central Google Scholar
Kinoshita, N. et al. Efficacy of combined orthokeratology and 0.01% atropine solution for slowing axial elongation in children with myopia: a 2-year randomised trial. Sci. Rep. 10 , 12750 (2020).
Clark, T. Y. & Clark, R. A. Atropine 0.01% eyedrops significantly reduce the progression of childhood myopia. J. Ocul. Pharmacol. Ther. 31 , 541–545 (2015). This study showed that atropine at 0.01% could significantly reduce the rate of myopic progression over 1 year in children with minimal side effects .
Anderle, R. & Ventruba, J. The current state of refractive surgery. Coll. Antropol. 37 (Suppl. 1), 237–241 (2013). This article provides an overall view of refractive surgery .
Wen, D. et al. Postoperative efficacy, predictability, safety, and visual quality of laser corneal refractive surgery: a network meta-analysis. Am. J. Ophthalmol. 178 , 65–78 (2017).
Kamiya, K. et al. A multicenter retrospective survey of refractive surgery in 78,248 eyes. J. Refract. Surg. 33 , 598–602 (2017).
Yuen, L. H. et al. A 10-year prospective audit of LASIK outcomes for myopia in 37,932 eyes at a single institution in Asia. Ophthalmology 117 , 1236–1244 e1231 (2010). This study demonstrates the efficacy, safety and predictability of LASIK .
Kezirian, G. M. & Stonecipher, K. G. Comparison of the IntraLase femtosecond laser and mechanical keratomes for laser in situ keratomileusis. J. Cataract Refract. Surg. 30 , 804–811 (2004).
Rosman, M. et al. Comparison of efficacy and safety of laser in situ keratomileusis using 2 femtosecond laser platforms in contralateral eyes. J. Cataract Refract. Surg. 39 , 1066–1073 (2013).
Zalentein, W. N., Tervo, T. M. & Holopainen, J. M. Seven-year follow-up of LASIK for myopia. J. Refract. Surg. 25 , 312–318 (2009).
Giri, P. & Azar, D. T. Risk profiles of ectasia after keratorefractive surgery. Curr. Opin. Ophthalmol. 28 , 337–342 (2017).
Wolle, M. A., Randleman, J. B. & Woodward, M. A. Complications of refractive surgery: ectasia after refractive surgery. Int. Ophthalmol. Clin. 56 , 127–139 (2016).
Shortt, A. J. & Allan, B. D. Photorefractive keratectomy (PRK) versus laser-assisted in-situ keratomileusis (LASIK) for myopia. Cochrane Database Syst. Rev. 2 , CD005135 (2006).
Matsumoto, J. C. & Chu, Y. S. Epi-LASIK update: overview of techniques and patient management. Int. Ophthalmol. Clin. 46 , 105–115 (2006).
Sia, R. K., Coe, C. D., Edwards, J. D., Ryan, D. S. & Bower, K. S. Visual outcomes after Epi-LASIK and PRK for low and moderate myopia. J. Refract. Surg. 28 , 65–71 (2012).
Kuryan, J., Cheema, A. & Chuck, R. S. Laser-assisted subepithelial keratectomy (LASEK) versus laser-assisted in-situ keratomileusis (LASIK) for correcting myopia. Cochrane Database Syst. Rev. 2 , CD011080 (2017).
Naderi, M., Jadidi, K., Mosavi, S. A. & Daneshi, S. A. Transepithelial photorefractive keratectomy for low to moderate myopia in comparison with conventional photorefractive keratectomy. J. Ophthalmic Vis. Res. 11 , 358–362 (2016).
Shortt, A. J., Allan, B. D. S. & Evans, J. R. Laser-assisted in-situ keratomileusis (LASIK) versus photorefractive keratectomy (PRK) for myopia. Cochrane Database Syst. Rev. 1 , CD005135 (2013).
Santhiago, M. R., Netto, M. V. & Wilson, S. E. Mitomycin C: biological effects and use in refractive surgery. Cornea 31 , 311–321 (2012).
Wallau, A. D. & Campos, M. Photorefractive keratectomy with mitomycin C versus LASIK in custom surgeries for myopia: a bilateral prospective randomized clinical trial. J. Refract. Surg. 24 , 326–336 (2008).
Agca, A. et al. Refractive lenticule extraction (ReLEx) through a small incision (SMILE) for correction of myopia and myopic astigmatism: current perspectives. Clin. Ophthalmol. 10 , 1905–1912 (2016). This article provides an overall view of SMILE .
Chan, C., Lawless, M., Sutton, G., Versace, P. & Hodge, C. Small incision lenticule extraction (SMILE) in 2015. Clin. Exp. Optom. 99 , 204–212 (2016).
Cai, W. T. et al. Dry eye and corneal sensitivity after small incision lenticule extraction and femtosecond laser-assisted in situ keratomileusis: a Meta-analysis. Int. J. Ophthalmol. 10 , 632–638 (2017).
Fernandez, J., Valero, A., Martinez, J., Pinero, D. P. & Rodriguez-Vallejo, M. Short-term outcomes of small-incision lenticule extraction (SMILE) for low, medium, and high myopia. Eur. J. Ophthalmol. 27 , 153–159 (2017).
Kim, J. R., Kim, B. K., Mun, S. J., Chung, Y. T. & Kim, H. S. One-year outcomes of small-incision lenticule extraction (SMILE): mild to moderate myopia vs. high myopia. BMC Ophthalmol. 15 , 59 (2015).
Osman, I. M., Helaly, H. A., Abdalla, M. & Shousha, M. A. Corneal biomechanical changes in eyes with small incision lenticule extraction and laser assisted in situ keratomileusis. BMC Ophthalmol. 16 , 123 (2016).
Guell, J. L., Morral, M., Kook, D. & Kohnen, T. Phakic intraocular lenses part 1: historical overview, current models, selection criteria, and surgical techniques. J. Cataract Refract. Surg. 36 , 1976–1993 (2010). This article provides an overall view of PIOL .
Lackner, B. et al. Long-term results of implantation of phakic posterior chamber intraocular lenses. J. Cataract Refract. Surg. 30 , 2269–2276 (2004).
Perez-Cambrodi, R. J., Piñero, D. P., Ferrer-Blasco, T., Cerviño, A. & Brautaset, R. The posterior chamber phakic refractive lens (PRL): a review. Eye 27 , 14–21 (2013).
Lee, J. et al. Long-term clinical results of posterior chamber phakic intraocular lens implantation to correct myopia. Clin. Exp. Ophthalmol. 44 , 481–487 (2016).
Guerra, M. G. et al. Phakic intraocular lens implantation: refractive outcome and safety in patients with anterior chamber depth between 2.8 and 3.0 versus >/=3.0 mm. Ophthalmic Res. 57 , 239–246 (2017).
AlSabaani, N. A. et al. Causes of phakic implantable collamer lens explantation/exchange at king khaled eye specialist hospital. Middle East Afr. J. Ophthalmol. 23 , 293–295 (2016).
Fernandes, P. et al. Implantable collamer posterior chamber intraocular lenses: a review of potential complications. J. Refract. Surg. 27 , 765–776 (2011).
Arne, J. L. Phakic intraocular lens implantation versus clear lens extraction in highly myopic eyes of 30- to 50-year-old patients. J. Cataract Refract. Surg. 30 , 2092–2096 (2004).
Nanavaty, M. A. & Daya, S. M. Refractive lens exchange versus phakic intraocular lenses. Curr. Opin. Ophthalmol. 23 , 54–61 (2012).
Basch, E. Patient-reported outcomes - harnessing patients’ voices to improve clinical care. N. Engl. J. Med. 376 , 105–108 (2017).
Sensaki, S. et al. An ecologic study of trends in the prevalence of myopia in Chinese adults in Singapore born from the 1920s to 1980s. Ann. Acad. Med. Singap. 46 , 229–236 (2017).
Coletta, N. J. & Watson, T. Effect of myopia on visual acuity measured with laser interference fringes. Vis. Res. 46 , 636–651 (2006).
Mitchell, P., Hourihan, F., Sandbach, J. & Wang, J. J. The relationship between glaucoma and myopia: the Blue Mountains eye study. Ophthalmology 106 , 2010–2015 (1999).
Polkinghorne, P. J. & Craig, J. P. Northern New Zealand rhegmatogenous retinal detachment study: epidemiology and risk factors. Clin. Exp. Ophthalmol. 32 , 159–163 (2004).
Praveen, M. R., Vasavada, A. R., Jani, U. D., Trivedi, R. H. & Choudhary, P. K. Prevalence of cataract type in relation to axial length in subjects with high myopia and emmetropia in an Indian population. Am. J. Ophthalmol. 145 , 176–181 (2008).
Tan, D., Tay, S. A., Loh, K. L. & Chia, A. Topical atropine in the control of myopia. Asia Pac. J. Ophthalmol. 5 , 424–428 (2016).
Lamoureux, E. L. et al. The impact of corrected and uncorrected refractive error on visual functioning: the Singapore Malay eye study. Invest. Ophthalmol. Vis. Sci. 50 , 2614–2620 (2009).
Kandel, H. et al. Uncorrected and corrected refractive error experiences of Nepalese adults: a qualitative study. Ophthalmic Epidemiol. 25 , 147–161 (2018).
Ayaki, M., Torii, H., Tsubota, K. & Negishi, K. Decreased sleep quality in high myopia children. Sci. Rep. 6 , 33902 (2016).
Rose, K. et al. Quality of life in myopia. Br. J. Ophthalmol. 84 , 1031–1034 (2000).
Gwiazda, J. Treatment options for myopia. Optom. Vis. Sci. 86 , 624–628 (2009).
Sutton, G., Lawless, M. & Hodge, C. Laser in situ keratomileusis in 2012: a review. Clin. Exp. Optom. 97 , 18–29 (2014).
Uusitalo, R. J., Aine, E., Sen, N. H. & Laatikainen, L. Implantable contact lens for high myopia. J. Cataract Refract. Surg. 28 , 29–36 (2002).
Markoulli, M. & Kolanu, S. Contact lens wear and dry eyes: challenges and solutions. Clin. Optom. 9 , 41–48 (2017).
Shtein, R. M. Post-LASIK dry eye. Expert Rev. Ophthalmol. 6 , 575–582 (2011).
Kandel, H., Khadka, J., Goggin, M. & Pesudovs, K. Impact of refractive error on quality of life: a qualitative study. Clin. Exp. Ophthalmol. 45 , 677–688 (2017).
Van Der Wees, P. J. et al. Integrating the use of patient-reported outcomes for both clinical practice and performance measurement: views of experts from 3 countries. Milbank Q. 92 , 754–775 (2014).
Pesudovs, K., Garamendi, E. & Elliott, D. B. The quality of life impact of refractive correction (QIRC) questionnaire: development and validation. Optom. Vis. Sci. 81 , 769–777 (2004).
Berry, S., Mangione, C. M., Lindblad, A. S. & McDonnell, P. J. Development of the national eye institute refractive error correction quality of life questionnaire: focus groups. Ophthalmology 110 , 2285–2291 (2003).
Vitale, S., Schein, O. D., Meinert, C. L. & Steinberg, E. P. The refractive status and vision profile: a questionnaire to measure vision-related quality of life in persons with refractive error. Ophthalmology 107 , 1529–1539 (2000).
Kandel, H., Khadka, J., Goggin, M. & Pesudovs, K. Patient-reported outcomes for assessment of quality of life in refractive error: a systematic review. Optom. Vis. Sci. 94 , 1102–1119 (2017). Comprehensive overview of current myopia-specific instruments and the shortcomings of each instrument, highlighting the current gap in myopia PROM assessment .
Cella, D., Gershon, R., Lai, J. S. & Choi, S. The future of outcomes measurement: item banking, tailored short-forms, and computerized adaptive assessment. Qual. Life Res. 16 (Suppl. 1), 133–141 (2007).
Gershon, R. C. Computer adaptive testing. J. Appl. Meas. 6 , 109–127 (2005).
Baumhauer, J. F. & Bozic, K. J. Value-based healthcare: patient-reported outcomes in clinical decision making. Clin. Orthop. Relat. Res. 474 , 1375–1378 (2016). This is a detailed look at how PROMs are becoming increasingly important in this era of value-based and personalized health care .
Hu, Y. et al. Association of age at myopia onset with risk of high myopia in adulthood in a 12-year follow-up of a Chinese cohort. JAMA Ophthalmol. https://doi.org/10.1001/jamaophthalmol.2020.3451 (2020).
Article PubMed PubMed Central Google Scholar
Chia, A. et al. Atropine for the treatment of childhood myopia: safety and efficacy of 0.5%, 0.1%, and 0.01% doses (atropine for the treatment of myopia 2). Ophthalmology 119 , 347–354 (2012).
Hyman, L. et al. Relationship of age, sex, and ethnicity with myopia progression and axial elongation in the correction of myopia evaluation trial. Arch. Ophthalmol. 123 , 977–987 (2005).
Zhao, J. et al. The progression of refractive error in school-age children: Shunyi district, China. Am. J. Ophthalmol. 134 , 735–743 (2002).
Chen, Y. et al. Contribution of genome-wide significant single nucleotide polymorphisms in myopia prediction: findings from a 10-year cohort of Chinese twin children. Ophthalmology 126 , 1607–1614 (2019).
Lin, H. et al. Prediction of myopia development among Chinese school-aged children using refraction data from electronic medical records: a retrospective, multicentre machine learning study. PLoS Med. 15 , e1002674 (2018).
Chen, Y., Zhang, J., Morgan, I. G. & He, M. Identifying children at risk of high myopia using population centile curves of refraction. PLoS ONE 11 , e0167642 (2016).
Chua, S. Y. et al. Age of onset of myopia predicts risk of high myopia in later childhood in myopic Singapore children. Ophthalmic Physiol. Opt. 36 , 388–394 (2016).
Zadnik, K. et al. Prediction of juvenile-onset myopia. JAMA Ophthalmol. 133 , 683–689 (2015).
Tideman, J. W. L. et al. Axial length growth and the risk of developing myopia in european children. Acta Ophthalmol. 96 , 301–309 (2018).
Verkicharla, P. K. et al. Development of the fitsight fitness tracker to increase time outdoors to prevent myopia. Transl. Vis. Sci. Technol. 6 , 20 (2017).
Wen, L. et al. An objective comparison of light intensity and near-visual tasks between rural and urban school children in China by a wearable device Clouclip. Transl. Vis. Sci. Technol. 8 , 15 (2019).
Lanca, C. & Saw, S. M. The association between digital screen time and myopia: a systematic review. Ophthalmic Physiol. Opt. 40 , 216–229 (2020).
Smith, E. L. III Prentice award lecture 2010: a case for peripheral optical treatment strategies for myopia. Optometry Vis. Sci. 88 , 1029–1044 (2011).
Download references
Acknowledgements
The authors thank Ian Morgan (Australian National University) for his tremendous help on preparing this manuscript. This study was supported by the Australian National Health and Medical Research Council (NHMRC) through project grant 1034329 and a Senior Research Fellowship (1138585 to P.N.B.); the National Medical Research Council (NRMC) Grant NMRC/CIRG/1446/2017 and A*STAR World Without Disease (Grant JRBMRR 151701) (S.-M.S. and C.L.); Clinician Scientist Award (Senior; NMRC/CSA-SI/0009/2016) (E.L.L.) and Transition Award (MOH-TA19may-0002) (R.M.); National Institutes of Health Grant EY-03611 and funds from the Brien Holden Vision Institute (E.L.S.); grants 81800860, 81422007 and 81371047 from the National Natural Science Foundation of China (X.Z.); Fight for Sight and Welsh Government Project Grant (Ref: 24WG201) (J.A.G.); and Fundamental Research Funds of the State Key Laboratory of Ophthalmology, the Research Accelerator Program at the University of Melbourne and the CERA Foundation in Australia (M.H.).
Author information
Authors and affiliations.
Department of Surgery in Ophthalmology, Faculty of Medicine, Dentistry and Health Sciences, The University of Melbourne, Royal Victorian Eye and Ear Hospital, Melbourne, Victoria, Australia
Paul N. Baird
Myopia Research Group, Singapore Eye Research Institute, Singapore, Singapore
Seang-Mei Saw & Carla Lanca
Duke-NUS Medical School, Singapore, Singapore
Seang-Mei Saw
Saw Swee Hock School of Public Health, National University of Singapore, Singapore, Singapore
School of Optometry & Vision Sciences, Cardiff University, Cardiff, UK
Jeremy A. Guggenheim
College of Optometry, University of Houston, Houston, TX, USA
Earl L. Smith III
School of Ophthalmology and Optometry, Eye Hospital, Wenzhou Medical University, Wenzhou, China
Xiangtian Zhou
Department of Ophthalmology and Visual Science, Tokyo Medical and Dental University, Tokyo, Japan
Kyoko-Ohno Matsui
Department of Ophthalmology, Kaohsiung Chang Gung Memorial Hospital; Chang Gung University College of Medicine, Taoyuan, Taiwan
Pei-Chang Wu
Brien Holden Vision Institute, Sydney, New South Wales, Australia
Padmaja Sankaridurg
Paediatric Ophthalmology Service, Singapore National Eye Centre, Singapore, Singapore
Audrey Chia
Refractive Surgery Department, Singapore National Eye Centre, Singapore, Singapore
Mohamad Rosman
Duke-NUS Medical School and Singapore Eye Research Institute, Singapore, Singapore
Mohamad Rosman, Ecosse L. Lamoureux & Ryan Man
Population Health Research Unit, Singapore Eye Research Institute, Singapore, Singapore
Ecosse L. Lamoureux & Ryan Man
Centre for Eye Research Australia, Ophthalmology, Department of Surgery, University of Melbourne, Melbourne, Victoria, Australia
Mingguang He
State Key Laboratory of Ophthalmology, Zhongshan Ophthalmic Center, Sun Yat-sen University, Guangzhou, China
You can also search for this author in PubMed Google Scholar
Contributions
Introduction (M.H., P.N.B. and J.A.G.); Epidemiology (S.-M.S., C.L., M.H., J.A.G. and P.N.B.); Mechanisms/Pathophysiology (M.H., P.N.B., J.A.G., E.L.S. and X.Z.); Diagnosis, screening and prevention (M.H. and K.-O.M.); Management (P.-C.W., P.S., A.C. and M.R.); Quality of life (E.L.L. and R.M.); Outlook (P.N.B., M.H. and J.A.G.); Overview of Primer (P.N.B. and M.H.).
Corresponding authors
Correspondence to Paul N. Baird or Mingguang He .
Ethics declarations
Competing interests.
The authors declare no competing interests.
Additional information
Peer review information.
Nature Reviews Disease Primers thanks C. Leung, F. Schaeffel, K. Tsubota, T. Young and the other, anonymous, reviewer(s) for their contribution to the peer review of this work.
Publisher’s note
Springer Nature remains neutral with regard to jurisdictional claims in published maps and institutional affiliations.
Placing a diffusing filter in front of the eye.
Image formed in front of retinal plane.
The distance from the anterior surface of the cornea to the retina.
Ability to focus on distant objects.
The development of the eye towards emmetropia, wherein the focus of distant objects is on the retina when the lens is in a relaxed state.
The ability of the eye to change its focus from near to far.
Blur resulting from the image formed behind the retinal plane.
A highly vascularized layer between the sclera and the retina.
Paralysis of the ciliary muscles of the eye.
The interior surface of the back of the eye, comprising the retina, choroid and sclera.
Examination to provide a wide view of the back of the eye using a beam of light and a hand-held lens.
Protrusion of a limited area of the posterior sclera.
The formation of new blood vessels in the choroid of the eye.
Rigid, gas-permeable lenses to flatten corneal curvature during overnight wear.
Sensitivity to light.
A laser emitting concentrated light in the ultraviolet region of the spectrum.
(PIOL). Silicon or plastic lenses inserted into a person’s eye to restore vision but leaves the eye’s natural lens intact.
Abnormal change in shape of the cornea.
A granular scarring of the corneal stroma due to inflammation.
A small piece of corneal or synthetic material with a precise shape and thickness implanted into the cornea in order to change the corneal curvature.
Infection of the interior of the eye.
Measurement of specific features that are common to the eye.
A psychometric model for analysing categorical data, typically in the form of a questionnaire.
Rights and permissions
Reprints and permissions
About this article
Cite this article.
Baird, P.N., Saw, SM., Lanca, C. et al. Myopia. Nat Rev Dis Primers 6 , 99 (2020). https://doi.org/10.1038/s41572-020-00231-4
Download citation
Accepted : 29 October 2020
Published : 17 December 2020
DOI : https://doi.org/10.1038/s41572-020-00231-4
Share this article
Anyone you share the following link with will be able to read this content:
Sorry, a shareable link is not currently available for this article.
Provided by the Springer Nature SharedIt content-sharing initiative
This article is cited by
Scleral remodeling during myopia development in mice eyes: a potential role of thrombospondin-1.
- Junhan Chen
- Shin-ichi Ikeda
- Toshihide Kurihara
Molecular Medicine (2024)
Comparison of macular changes and visual outcomes between femtosecond laser-assisted cataract surgery and conventional phacoemulsification surgery for high myopic cataract patients
- Xuemei Liang
BMC Ophthalmology (2024)
The influence of the environment and lifestyle on myopia
- Sayantan Biswas
- Antonio El Kareh
- Raymond P. Najjar
Journal of Physiological Anthropology (2024)
Association between weight-adjusted waist index and myopia in adolescents and young adults: results from NHANES 1999–2008
- Wen Bin Wei
Associations between corneal curvature and other anterior segment biometrics in young myopic adults
Scientific Reports (2024)
Quick links
- Explore articles by subject
- Guide to authors
- Editorial policies
Sign up for the Nature Briefing newsletter — what matters in science, free to your inbox daily.

- Introduction
- Conclusions
- Article Information
HAL indicates spectacle lenses with highly aspherical lenslets; PAL, progressive addition lenses; SAL, spectacle lenses with slightly aspherical lenslets; SVL, single-vision spectacle lenses.
Error bars represent standard errors of the mean. HAL indicates spectacle lenses with highly aspherical lenslets; SAL, spectacle lenses with slightly aspherical lenslets; SVL, single-vision spectacle lenses.
Trial Protocol
Standard Operating Procedures
Statistical Analysis Plan
Data Sharing Statement
- JAMA Network Articles of the Year 2022 JAMA Medical News & Perspectives December 27, 2022 This Medical News article is our annual roundup of the top-viewed articles from all JAMA Network journals. Melissa Suran, PhD, MSJ
- Clinical Relevance of Myopia Control With Specialized Spectacles JAMA Ophthalmology Invited Commentary May 1, 2022 David C. Musch, PhD, MPH; Steven M. Archer, MD
See More About
Select your interests.
Customize your JAMA Network experience by selecting one or more topics from the list below.
- Academic Medicine
- Acid Base, Electrolytes, Fluids
- Allergy and Clinical Immunology
- American Indian or Alaska Natives
- Anesthesiology
- Anticoagulation
- Art and Images in Psychiatry
- Artificial Intelligence
- Assisted Reproduction
- Bleeding and Transfusion
- Caring for the Critically Ill Patient
- Challenges in Clinical Electrocardiography
- Climate and Health
- Climate Change
- Clinical Challenge
- Clinical Decision Support
- Clinical Implications of Basic Neuroscience
- Clinical Pharmacy and Pharmacology
- Complementary and Alternative Medicine
- Consensus Statements
- Coronavirus (COVID-19)
- Critical Care Medicine
- Cultural Competency
- Dental Medicine
- Dermatology
- Diabetes and Endocrinology
- Diagnostic Test Interpretation
- Drug Development
- Electronic Health Records
- Emergency Medicine
- End of Life, Hospice, Palliative Care
- Environmental Health
- Equity, Diversity, and Inclusion
- Facial Plastic Surgery
- Gastroenterology and Hepatology
- Genetics and Genomics
- Genomics and Precision Health
- Global Health
- Guide to Statistics and Methods
- Hair Disorders
- Health Care Delivery Models
- Health Care Economics, Insurance, Payment
- Health Care Quality
- Health Care Reform
- Health Care Safety
- Health Care Workforce
- Health Disparities
- Health Inequities
- Health Policy
- Health Systems Science
- History of Medicine
- Hypertension
- Images in Neurology
- Implementation Science
- Infectious Diseases
- Innovations in Health Care Delivery
- JAMA Infographic
- Law and Medicine
- Leading Change
- Less is More
- LGBTQIA Medicine
- Lifestyle Behaviors
- Medical Coding
- Medical Devices and Equipment
- Medical Education
- Medical Education and Training
- Medical Journals and Publishing
- Mobile Health and Telemedicine
- Narrative Medicine
- Neuroscience and Psychiatry
- Notable Notes
- Nutrition, Obesity, Exercise
- Obstetrics and Gynecology
- Occupational Health
- Ophthalmology
- Orthopedics
- Otolaryngology
- Pain Medicine
- Palliative Care
- Pathology and Laboratory Medicine
- Patient Care
- Patient Information
- Performance Improvement
- Performance Measures
- Perioperative Care and Consultation
- Pharmacoeconomics
- Pharmacoepidemiology
- Pharmacogenetics
- Pharmacy and Clinical Pharmacology
- Physical Medicine and Rehabilitation
- Physical Therapy
- Physician Leadership
- Population Health
- Primary Care
- Professional Well-being
- Professionalism
- Psychiatry and Behavioral Health
- Public Health
- Pulmonary Medicine
- Regulatory Agencies
- Reproductive Health
- Research, Methods, Statistics
- Resuscitation
- Rheumatology
- Risk Management
- Scientific Discovery and the Future of Medicine
- Shared Decision Making and Communication
- Sleep Medicine
- Sports Medicine
- Stem Cell Transplantation
- Substance Use and Addiction Medicine
- Surgical Innovation
- Surgical Pearls
- Teachable Moment
- Technology and Finance
- The Art of JAMA
- The Arts and Medicine
- The Rational Clinical Examination
- Tobacco and e-Cigarettes
- Translational Medicine
- Trauma and Injury
- Treatment Adherence
- Ultrasonography
- Users' Guide to the Medical Literature
- Vaccination
- Venous Thromboembolism
- Veterans Health
- Women's Health
- Workflow and Process
- Wound Care, Infection, Healing
Others Also Liked
- Download PDF
- X Facebook More LinkedIn
Bao J , Huang Y , Li X, et al. Spectacle Lenses With Aspherical Lenslets for Myopia Control vs Single-Vision Spectacle Lenses : A Randomized Clinical Trial . JAMA Ophthalmol. 2022;140(5):472–478. doi:10.1001/jamaophthalmol.2022.0401
Manage citations:
© 2024
- Permissions
Spectacle Lenses With Aspherical Lenslets for Myopia Control vs Single-Vision Spectacle Lenses : A Randomized Clinical Trial
- 1 Eye Hospital and School of Ophthalmology and Optometry, Wenzhou Medical University, Wenzhou, Zhejiang, China
- 2 National Clinical Research Center for Ocular Diseases, Wenzhou, Zhejiang, China
- 3 Wenzhou Medical University–Essilor International Research Center (WEIRC), Wenzhou Medical University, Wenzhou, Zhejiang, China
- 4 R&D AMERA, Essilor International, Singapore, Singapore
- Invited Commentary Clinical Relevance of Myopia Control With Specialized Spectacles David C. Musch, PhD, MPH; Steven M. Archer, MD JAMA Ophthalmology
- Medical News & Perspectives JAMA Network Articles of the Year 2022 Melissa Suran, PhD, MSJ JAMA
Question What is the efficacy of spectacle lenses with highly aspherical lenslets and slightly aspherical lenslets compared with conventional single-vision spectacle lenses in controlling myopia progression throughout 2 years?
Findings In this randomized clinical trial of 157 children, the amount of myopia progression and axial length increase was significantly less in both the highly aspherical lenslets group and the slightly aspherical lenslets group vs the single-vision spectacle lenses group at 24 months.
Meaning The findings of this study suggest that a higher asphericity of lenslets may be associated with more effective myopia control.
Importance Reducing myopia progression can reduce the risk of associated ocular pathologies.
Objective To evaluate whether spectacle lenses with higher lenslet asphericity have a higher myopia control efficacy throughout 2 years.
Design, Setting, and Participants This double-masked randomized clinical trial was conducted between July 2018 and October 2020 at the Eye Hospital of Wenzhou Medical University in Wenzhou, China. Children aged 8 to 13 years with a cycloplegic spherical equivalent refraction (SER) of −0.75 D to −4.75 D and astigmatism with less than −1.50 D were recruited. A data and safety monitoring committee reviewed findings from a planned interim analysis in 2019.
Interventions Participants were randomly assigned in a 1:1:1 ratio to receive spectacle lenses with highly aspherical lenslets (HAL), spectacle lenses with slightly aspherical lenslets (SAL), or single-vision spectacle lenses (SVL).
Main Outcome and Measures Two-year changes in SER and axial length and their differences between groups.
Results Of 157 participants who completed each visit (mean [SD] age, 10.4 [1.2] years), 54 were analyzed in the HAL group, 53 in the SAL group, and 50 in the SVL group. Mean (SE) 2-year myopia progression in the SVL group was 1.46 (0.09) D. Compared with SVL, the mean (SE) change in SER was less for HAL (by 0.80 [0.11] D) and SAL (by 0.42 [0.11] D; P ≤ .001). The mean (SE) increase in axial length was 0.69 (0.04) mm for SVL. Compared with SVL, increase in axial length was slowed by a mean (SE) of 0.35 (0.05) mm for HAL and 0.18 (0.05) mm for SAL ( P ≤ .001). Compared with SVL, for children who wore HAL at least 12 hours every day, the mean (SE) change in SER was slowed by 0.99 (0.12) D, and increase in axial length slowed by 0.41 (0.05) mm.
Conclusions and Relevance In this study, HAL and SAL reduced the rate of myopia progression and axial elongation throughout 2 years, with higher efficacy for HAL. Longer wearing hours resulted in better myopia control efficacy for HAL.
Trial Registration Chinese Clinical Trial Registry Identifier: ChiCTR1800017683
The projected 2050 prevalence rates of myopia and high myopia are alarming. 1 Myopia control interventions have been used for many years to reduce the severity of myopia and decrease the risk of associated ocular pathologies. 2 Increasing evidence suggests that specifically designed optical interventions such as spectacle lenses, soft contact lenses, and orthokeratology slow myopia progression in children. 3 - 5 The common features of these myopia optical interventions are to provide central correction for distance vision and correct peripheral retinal defocus or induce peripheral myopic retinal defocus simultaneously. Peripheral visual signals have been found to dominate central refractive development, 6 and the effect of peripheral myopic retinal defocus was found to provide myopia control signals. 7 - 9 Furthermore, several studies have shown a positive dose-response relationship between the efficacy of optical interventions and parameters such as addition power in multifocal soft contact lenses 10 and spectacle lenses 11 , 12 and in the wearing time of multifocal soft contact lenses. 13 This study evaluates novel spectacle lenses with aspherical lenslets and explores the effect of lenslet asphericity on myopia control efficacy. The results from the first-year interim analysis showed that spectacle lenses with highly aspherical lenslets (HAL) and spectacle lenses with slightly aspherical lenslets (SAL) were effective in slowing myopia progression. Moreover, a dose-dependent response was observed, as HAL had significantly better myopia control effect than SAL. 14
We aimed to evaluate whether spectacle lenses with aspherical lenslets still slow myopia progression over 2 years and whether the level of lenslet asphericity would still affect myopia control efficacy in a dose-dependent manner.
This 2-year, double-masked, 3-group, parallel randomized, single-center clinical trial comparing the effect of spectacle lenses with aspherical lenslets with single-vision spectacle lenses (SVL) in slowing myopia progression among children was conducted in the Eye Hospital of Wenzhou Medical University in Wenzhou, China, from July 18, 2018, to October 7, 2020. The study design has been described previously. 14 The trial protocol, standard operating procedures, and statistical analysis plan are available in Supplement 1 , Supplement 2 , and Supplement 3 , respectively. Children were randomly assigned to wear HAL, SAL, or SVL based on their baseline right eye cycloplegic spherical equivalent refraction (SER), age, and sex. Assignment with online software (Randola 15 ) was performed by a specified person who had no interaction with the participants and stored on the website that required login credentials. The same person would pack the lenses according to the randomization in an unlabeled envelope with participant identification as the only identification for dispensing. Masking was maintained for refraction and axial length measurements for dispensing personnel and examiners, participants, and parents or guardians. However, it was not entirely possible to mask HAL and SAL lenses from SVL because lenslets were visible under certain lighting conditions. However, complete masking was possible between HAL and SAL. Written informed consent was obtained from the parent or guardian, and written assent was obtained from the study participant. All study participants were given a pair of myopia-control spectacles on completion of the study. A data and safety monitoring committee reviewed the trial data for participant safety. The ethics committee of Eye Hospital of Wenzhou Medical University approved the clinical trial on July 17, 2018, and the trial was registered with the Chinese Clinical Trial Registry. All procedures adhered to the tenets of the Declaration of Helsinki. 16
The first participant was enrolled on August 11, 2018. Chinese children aged 8 to 13 years who had myopia, SER from −0.75 diopters (D) to −4.75 D, astigmatism of 1.50 D cylinder or less, anisometropia of 1.00 D or less, and best-corrected visual acuity of 0.05 logMAR or better in each eye were randomized at a 1:1:1 ratio to wear HAL, SAL, or SVL. No participants had a history of myopia control or any ocular or systemic issues that could affect vision outcomes.
A detailed description of this study device can be found elsewhere. 14 The primary outcomes were changes in SER and axial length. The parameter measurement procedures followed those for the 1-year outcomes of HAL and SAL. 14 SER and axial length were measured every 6 months. SER was measured by the mode of 10 measurements using a Topcon KR-800 (Topcon Corporation), which was acquired at least 30 minutes after installing 2 drops of cyclopentolate, 1%, administered 5 minutes apart. Axial length was calculated as the mean of 5 measurements obtained using a Lenstar LS 900 instrument (Haag-Streit AG).
The secondary outcomes presented in this report were lens wearing hours assessed by 6-month questionnaires with self-reported wearing times per day, every day per week (Monday to Sunday).
Based on previous findings, 12 , 17 , 18 we anticipated a mean (SD) SER progression of 1.50 (0.75) D and converted axial length progression of 0.6 (0.02) mm in the control group throughout a 2-year period. We wanted to identify a 33% reduction in the amount of SER and axial length progression for treatment groups compared with the control group. Fifty participants were required in each group for an α level of .05 (2-tailed), a power of 90%, and a dropout rate of 10%. With an interim analysis at 1 year, the α level was adjusted to .029 based on the Pocock method for 2-year outcomes of SER and axial length.
All data from participants who had complete 2-year follow-up records were analyzed. The mean values for ocular parameters measured in the right eye were used because there was a high correlation between the 2 eyes in SER ( r = 0.74, P < .001) and axial length ( r = 0.87, P < .001). The changes in SER and axial length from baseline are presented as mean (SE). χ 2 Test and analysis of variance with post hoc Bonferroni test were used to assess intergroup differences in categorical and continuous variables, respectively. Our analysis was performed using complete case data without imputation for missing data and dropouts. We undertook analyses using linear mixed model, adjusted for baseline age, sex, SER, axial length, age at myopia onset, and number of parents with myopia to evaluate the treatment effect. The mean daily lens wearing hours were calculated based on the mean daily lens wearing hours over the four 6-month periods. The effect of wearing time was analyzed categorically. Full-time wearers were defined as children who reported wearing their study devices for at least 12 hours every day. Part-time wearers were defined as nonfull-time wearers. SPSS statistical software version 24 (IBM) was used for data analysis. Two-sided P values of less than .05 were considered statistically significant.
One hundred seventy children with a mean (SD) age of 10.4 (1.2) years, ranging from 8 to 13 years, were recruited and randomized among the HAL (n = 58), SAL (n = 57), and SVL (n = 55) groups ( Figure 1 ). Only 167 were dispensed with the study equipment. Three children discontinued the study: 1 presented with intermittent exotropia not apparent during screening, 1 belatedly reported history of using myopia control, and 1 dropped out before test lenses were dispensed. After 2 years, 157 participants had completed all their visits; among children who did not attend their follow-up appointments, 2 (3.6%), 3 (5.4%), and 4 (7.3%) were in the HAL, SAL, and SVL groups, respectively. The reasons for dropout were not related to study devices. The demographic and ocular characteristics of each group at baseline are shown in Table 1 .
The 2-year means (SEs) for myopia progression were −0.66 (0.09) D, −1.04 (0.06) D, and −1.46 (0.09) D in the HAL, SAL, and SVL groups, respectively ( Figure 2 ). Significant differences were found among treatment groups ( F 2,154 = 25.80; P < .001). The HAL and SAL groups had less SER progression by a mean (SE) of 0.80 (0.11) D (95% CI, 0.53-1.07; P < .001) and 0.42 (0.11) D (95% CI, 0.15-0.70; P = .001), respectively, than the SVL group. In addition, the HAL group had less SER progression than the SAL group, with a mean (SE) difference of 0.38 (0.11) D (95% CI, 0.11-0.64; P = .002).
In the linear mixed model analysis, baseline age (95% CI, 0.004-0.128; P = .04) was directly, significantly associated with SER progression. The model-adjusted mean (SE) changes in SER were −0.68 (0.07) D, −1.04 (0.08) D, and −1.45 (0.08) D for HAL, SAL, and SVL, respectively, with a significant effect of lens design ( F 6,154 = 7.88; P < .001) on SER. Compared with SVL, the adjusted differences in mean (SE) SER were 0.77 (0.11) D (95% CI, 0.51-1.04; P < .001) and 0.42 (0.11) D (95% CI, 0.15-0.68; P = .001) in the HAL and SAL groups, respectively, and 0.36 (0.11) D (95% CI, 0.10-0.62; P = .003) between the HAL and SAL groups.
The mean (SE) increases in axial length over 2 years were 0.34 (0.03) mm, 0.51 (0.04) mm, and 0.69 (0.04) mm in the HAL, SAL, and SVL groups, respectively ( Figure 2 ). As with SER, there was a significant difference ( F 2,154 = 24.98; P < .001) in axial length increase among treatment groups. Compared with the SVL group, the HAL and SAL groups had reduced mean (SE) axial length elongation by 0.35 (0.05) mm (95% CI, 0.23-0.47; P < .001) and 0.18 (0.05) mm (95% CI, 0.06-0.30; P = .001), respectively. Furthermore, HAL had less axial length elongation than SAL by a mean (SE) of 0.17 (0.05) mm (95% CI, 0.05-0.29; P = .002).
In the linear mixed model analysis, baseline age (95% CI, −0.04 to −0.01; P = .002) and age at myopia onset (95% CI, 0.003-0.032; P = .02) were significantly associated with axial length elongation. After adjustment, the means (SEs) for change in axial length were 0.35 (0.03) mm, 0.50 (0.03) mm, and 0.68 (0.03) mm for HAL, SAL, and SVL, respectively, with 0.34 (0.05) mm (95% CI, 0.23-0.45; P < .001) and 0.18 (0.05) mm (95% CI, 0.07-0.30; P < .001) reductions in axial length elongation in the HAL and SAL groups compared with SVL. The HAL group had less axial length increase than SAL by a mean (SE) of 0.16 (0.05) mm (95% CI, 0.05-0.27; P = .002). As with SER, there was a significant effect of lens design ( F 6,154 = 8.41; P < .001) in axial length increase among treatment groups throughout 2 years.
During the second year, HAL still slowed myopia progression compared with SVL (mean [SE] for HAL, SER: −0.39 [0.05] D; P < .001; axial length, 0.21 [0.02] mm; P < .001). However, there were no differences in SER and axial length change observed between the SAL and SVL groups during the second year (mean [SE] for SER, SAL: −0.57 [0.05] D; SVL: −0.64 [0.05 D]; P = .90; axial length, SAL: 0.27 [0.02] mm; SVL: 0.32 [0.02] mm; P = .12). Thus, compared with SVL, SAL slowed myopia progression of children mainly during the first year.
The mean daily wearing time throughout 2 years was similar among the groups, with mean (SE) durations of 13.4 (0.29) hours, 13.4 (0.24) hours, and 13.9 (0.24) hours for HAL, SAL, and SVL, respectively ( F 2,154 = 1.08; P = .34). The mean wearing hours increased throughout the study from 13.1 hours in the first year to 14.0 hours in the second year (95% CI, 0.47-1.17; P < .001), with 61% (n = 96) of children who were full-time wearers in the first year to 89% (n = 139) who were full-time wearers in the second year ( P < .001). Therefore, we analyzed the myopia control efficacy based on the first-year full and part-time categories. The SER progression in the SAL and SVL groups was similar for full-time wearers and part-time wearers but was lower for full-time wearers in the HAL group (mean [SE] difference, 0.45 [0.16] D; P = .01; Table 2 ). Axial length elongation for full-time wearers was similar to that among part-time wearers in the SAL and SVL groups but was lower for full-time wearers in the HAL group (mean [SE] difference, 0.15 [0.07] mm; P = .03; Table 2 ). For full-time HAL wearers, the mean (SE) 2-year treatment effect compared with SVL group was 0.99 (0.12) D for SER and 0.41 (0.05) mm for axial length, whereas for HAL part-time wearers, it was 0.54 (0.15) D for SER and 0.26 (0.07) mm for axial length.
None of the adverse events reported were severe or resulted in study discontinuation. Two events were mild and unrelated to the study device and resolved with no reported loss of best-corrected visual acuity. One SVL wearer had punctate corneal epithelial defects, and the other SAL wearer was hit by a ball, causing the spectacle frame to break and the participant’s face to be bruised. There was no significant difference in adverse events between treatment groups ( P = .59).
In this 2-year randomized clinical trial, HAL slowed myopia progression by 0.80 D (55%) and increase in axial length by 0.35 mm (51%) compared with SVL. Compared with SAL, HAL slowed myopia progression by 0.38 D (37%) and axial length by 0.17 mm (33%). This outcome is well in line with the first-year interim analysis, 14 and it confirms a positive dose-response relationship between myopia control efficacy and lenslet asphericity. The dose-dependent effect of optical interventions in minimizing lens-induced myopia in animal studies was attributed mainly to lens design features such as the amount and area of lens addition, 19 , 20 peripheral defocus, and lens asphericity. 21 , 22 This dose-dependent relationship was also found in human clinical studies for ophthalmic lenses with higher addition 10 , 12 and contact lenses with higher asphericity. 23 Compared with SVL, during the second year, HAL remained effective in slowing myopia progression. This was not the case for SAL that slowed myopia progression of children mainly during the first year. This is similar to the previous myopia control study using progressive addition lenses. 24 It may be speculated that there is also a dose-response relationship between the duration of myopia control efficacy and lenslet asphericity. Therefore, it will be interesting to evaluate whether myopia control efficacy of HAL extends beyond 2 years.
Bifocal, prismatic bifocal spectacle lenses and defocus incorporated multiple segments (DIMS) spectacle lenses have also shown clinically significant myopia control results in Chinese children over the same duration as our study. 25 , 26 Throughout 2 years, the myopia control efficacy for HAL (0.80 D) and prismatic bifocal lenses (0.85 D) were comparable, while SAL (0.42 D), bifocal lenses (0.59 D), and DIMS (0.44 D) had lower myopia control efficacy. In terms of reducing axial elongation, HAL (0.35 mm) and DIMS (0.34 mm) had higher efficacy than SAL (0.18 mm) and bifocal and prismatic bifocal lenses (0.21 mm). Differences in myopia control efficacy could be linked to differences in lens designs, namely, the concentric ring configuration with aspherical lenslets (this study), honeycomb configuration with spherical lenslets (DIMS), 27 or increase in power over the lower part of the lens (bifocal and prismatic bifocal lenses). Another reason might be related to the control groups. Myopia progression rates among SVL wearers were −1.46 D in the present study and −1.55 D in the study by Cheng et al, 25 which are consistent with previous findings in Chinese children with myopia 28 , 29 while in the DIMS study, children wearing SVL progressed significantly less (−0.85 D).
The effect of wearing time on myopia control efficacy of HAL also indicated a similar dose-response relationship, increasing myopia control efficacy to 0.99 D (67%) and 0.41 mm (60%) for full-time wearers (at least 12 hours per day). A better myopia control effect with increased wearing time was also found in a study using defocus incorporated soft contact lenses. 13 However, no correlation between lens wearing time and myopia control efficacy was found in the study using DIMS spectacle lenses. 26 In the DIMS study, participants wore their study devices constantly for more than 15 hours per day. 26 In contrast, 39% of participants in this study wore their spectacles for less than 12 hours every day in the first year and 11% in the second year. Throughout 2 years, myopia progression and axial elongation were significantly lower among full-time wearers than among part-time wearers in the HAL group but not in the SVL or SAL group. As such, full-time wearing of myopia control intervention should be recommended for better outcome.
The first notable strength of this study is its low dropout rate of 6.5%. Second, the recruitment duration was short (approximately 2 months), with very punctual follow-ups (except the 18-month visit). This minimized the possible influence of confounding factors in the study, such as seasonal effects on myopia progression. 30
Nevertheless, there were some limitations to this study. First, this study was conducted with a strictly Chinese cohort in Wenzhou, China. According to the myopia screening of school-aged children (7 to 18 years) from more than 1000 elementary and high schools of Wenzhou in 2020, the overall myopia prevalence and high myopia prevalence were 59.35% and 4.99%, respectively, 31 which was comparable with the findings of 2 recent studies in China. 32 , 33 The current study sample could represent school-aged children in China but does not necessarily represent children with myopia globally. Second, the precision of the questionnaire may not reflect actual wearing hours. An objective measure of wearing time would be preferred. Third, the 18-month visit was delayed by a mean of 3 weeks. As a result of COVID-19 causing an unprecedented global pandemic, a lockdown was imposed for 3 weeks in February 2020. Children did not physically attend school for 4 months from February to the end of May 2020. 34 Other than the delay during the 18-month visit, COVID-19 did not have any major impact on the conduct of the study. The 24-month visit was conducted according to the investigation plan. Lastly, outdoor time was not measured and it may affect myopia progression in the study.
In children with myopia, wearing HAL significantly reduced the rate of myopia progression and eye growth over 2 years compared with SAL and SVL. This study demonstrated a dose-dependent effect, with higher lenslet asphericity having greater myopia control efficacy. The full-time wearing of HAL increased myopia control efficacy to 0.99 D (67%) for SER and 0.41 mm (60%) for axial length.
Accepted for Publication: February 1, 2022.
Published Online: March 31, 2022. doi:10.1001/jamaophthalmol.2022.0401
Corresponding Author: Hao Chen, MD, OD, Eye Hospital and School of Ophthalmology and Optometry, Wenzhou Medical University, 270 Xueyuan Rd, Wenzhou 325027, China ( [email protected] ).
Open Access: This is an open access article distributed under the terms of the CC-BY License . © 2022 Bao J et al. JAMA Ophthalmology .
Author Contributions: Dr Chen had full access to all of the data in the study and takes responsibility for the integrity of the data and the accuracy of the data analysis.
Concept and design: Bao, Yang, Spiegel, Drobe, Chen.
Acquisition, analysis, or interpretation of data: All authors.
Drafting of the manuscript: Bao, Yang, Zhou, Wu, Wang, Y. Li, Lim, Spiegel, Drobe.
Critical revision of the manuscript for important intellectual content: Bao, Huang, X. Li, Yang, Spiegel, Drobe, Chen.
Statistical analysis: Bao, Huang, X. Li, Yang, Spiegel, Drobe.
Obtained funding: Bao, Drobe, Chen.
Administrative, technical, or material support: Yang, Lim.
Supervision: Bao, Drobe, Chen.
Conflict of Interest Disclosures: Dr Drobe reported pending patents for WO2019166653, WO2019166654, and WO2019166655 (co-inventor). Drs Yang, Spiegel, and Drobe and Ms Lim are employees of Essilor International, which sells lenses with highly aspherical lenslets design and supplied the study device. No other disclosures were reported.
Funding/Support: This study was supported in part by the International S&T Cooperation Program of China (grant 2014DFA30940) and the collaborative research project with Essilor International (Wenzhou Medical University grants 95013006, 95016010, and 95020005).
Role of the Funder/Sponsor: Essilor International participated in the study design, management, analysis, and interpretation of the data; preparation, review, or approval of the manuscript; and decision to submit the manuscript for publication.
Data Sharing Statement: See Supplement 4 .
- Register for email alerts with links to free full-text articles
- Access PDFs of free articles
- Manage your interests
- Save searches and receive search alerts

- For Authors
- Editorial Board
- Journals Home
To View More...
Purchase this article with an account.
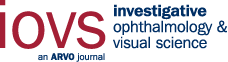
- Acknowledgments
- Nina Tahhan Brien Holden Vision Institute, Sydney Australia School of Optometry and Vision Science, Faculty of Medicine, University of New South Wales, Sydney, New South Wales, Australia
- James S. Wolffsohn College of Health & Life Sciences, Aston University, Birmingham, United Kingdom
- Padmaja Sankaridurg Brien Holden Vision Institute, Sydney Australia School of Optometry and Vision Science, Faculty of Medicine, University of New South Wales, Sydney, New South Wales, Australia
- Jost B. Jonas Department of Ophthalmology, Medical Faculty Mannheim, Heidelberg University, Mannheim, Germany Institute of Molecular and Clinical Ophthalmology Basel, Basel, Switzerland
- Mark A. Bullimore University of Houston, College of Optometry, Houston, Texas, United States
- Ian Flitcroft Centre for Eye Research Ireland, School of Physics and Clinical and Optometric Sciences, Technological University Dublin, Dublin, Ireland Department of Ophthalmology, Children's Health Ireland at Temple Street Hospital, Dublin, Ireland
- Lisa A. Ostrin University of Houston, College of Optometry, Houston, Texas, United States
- Christine Wildsoet UC Berkeley Wertheim School Optometry & Vision Science, Berkeley, California, United States
- Serge Resnikoff Brien Holden Vision Institute, Sydney Australia School of Optometry and Vision Science, Faculty of Medicine, University of New South Wales, Sydney, New South Wales, Australia
- Full Article
This feature is available to authenticated users only.
Nina Tahhan , James S. Wolffsohn , Padmaja Sankaridurg , Jost B. Jonas , Mark A. Bullimore , Ian Flitcroft , Lisa A. Ostrin , Christine Wildsoet , Serge Resnikoff; Editorial: International Myopia Institute White Paper Series 2023. Invest. Ophthalmol. Vis. Sci. 2023;64(6):1. https://doi.org/10.1167/iovs.64.6.1 .
Download citation file:
- Ris (Zotero)
- Reference Manager
© ARVO (1962-2015); The Authors (2016-present)
- Get Permissions
- Supplements

Supplemental Links
Related articles, from other journals, related topics.
- Visual Psychophysics and Physiological Optics
This PDF is available to Subscribers Only
You must be signed into an individual account to use this feature.
Log in using your username and password
- Search More Search for this keyword Advanced search
- Latest content
- Current issue
- Browse by collection
- BMJ Journals More You are viewing from: Google Indexer
You are here
- Online First
- Advances in myopia control strategies for children
- Article Text
- Article info
- Citation Tools
- Rapid Responses
- Article metrics

- Xiu Juan Zhang 1 ,
- Ebenezer Zaabaar 1 ,
- Amanda Nicole French 2 ,
- Fang Yao Tang 1 ,
- http://orcid.org/0000-0002-7760-6882 Ka Wai Kam 1 , 3 ,
- http://orcid.org/0000-0003-4407-6907 Clement C. Tham 1 , 3 , 4 , 5 , 6 , 7 ,
- http://orcid.org/0000-0003-3500-5840 Li Jia Chen 1 , 3 , 4 , 5 , 7 ,
- Chi Pui Pang 1 , 5 , 7 ,
- http://orcid.org/0000-0002-2156-1486 Jason C. Yam 1 , 3 , 4 , 6 , 7
- 1 Department of Ophthalmology and Visual Sciences , The Chinese University of Hong Kong , Hong Kong SAR , China
- 2 Discipline of Orthoptics , University of Sydney , Sydney , New South Wales , Australia
- 3 Department of Ophthalmology and Visual Sciences , The Prince of Wales Hospital , Hong Kong SAR , China
- 4 Hong Kong Eye Hospital , Hong Kong SAR , China
- 5 Lam Kin Chung. Jet King-Shing Ho Glaucoma Treatment and Research Centre, Department of Ophthalmology and Visual Sciences , The Chinese University of Hong Kong , Hong Kong SAR , China
- 6 Department of Ophthalmology , Hong Kong Children Hospital , Hong Kong SAR , China
- 7 Hong Kong Hub of Paediatric Excellence , The Chinese University of Hong Kong , Hong Kong SAR , China
- Correspondence to Dr Jason C. Yam, Department of Ophthalmology and Visual Sciences, The Chinese University of Hong Kong, Hong Kong SAR, China; yamcheuksing{at}cuhk.edu.hk
Myopia has long been a global threat to public health. Timely interventions are likely to reduce the risk of vision-threatening complications. There are both established and rapidly evolving therapeutic approaches to slow myopia progression and/or delay its onset. The effective methods for slowing myopia progression include atropine eye-drops, defocus incorporated multiple segments (DIMS) spectacle lenses, spectacle lenses with highly aspherical lenslets target (HALT), diffusion optics technology (DOT) spectacle lenses, red light therapy (RLT), multifocal soft contact lenses and orthokeratology. Among these, 0.05% atropine, HALT lenses, RLT and +3.00 peripheral addition soft contact lenses yield over 60% reduction in myopia progression, whereas DIMS, DOT and MiSight contact lenses demonstrate at least 50% myopia control efficacy. 0.05% atropine demonstrates a more optimal balance of efficacy and safety than 0.01%. The efficacy of 0.01% atropine has not been consistent and requires further validation across diverse ethnicities. Combining atropine 0.01% with orthokeratology or DIMS spectacles yields better outcomes than using these interventions as monotherapies. Increased outdoor time is an effective public health strategy for myopia prevention while recent studies suggest that 0.05% low-concentration atropine and RLT therapy have promising potential as clinical myopia prevention interventions for high-risk groups. Myopia control spectacle lenses, being the least invasive, are safe for long-term use. However, when considering other approaches, it is essential to ensure proper instruction and regular follow-ups to maintain safety and monitor any potential complications. Ultimately, significant advances have been made in myopia control strategies, many of which have shown meaningful clinical outcomes. However, regular use and adequate safety monitoring over extended durations are imperative to foster confidence that can only come from extensive clinical experience.
- Optics and Refraction
- Child health (paediatrics)
- Epidemiology
Data availability statement
All data relevant to the study are included in the article or uploaded as online supplemental information. Data sharing not applicable as no datasets generated and/or analysed for this study.
https://doi.org/10.1136/bjo-2023-323887
Statistics from Altmetric.com
Request permissions.
If you wish to reuse any or all of this article please use the link below which will take you to the Copyright Clearance Center’s RightsLink service. You will be able to get a quick price and instant permission to reuse the content in many different ways.
Introduction
Myopia has long been a global threat to public health with increasing prevalence across many regions in past decades, especially in East Asia. 1 2 It has been predicted that around half of the world’s population will become myopic and 1/10th highly myopic by the year 2050. 3 While myopia can be optically corrected, such corrections cannot address the core issue, the abnormal elongation of the eyeball. The excessive globe elongation places myopic individuals at a higher risk of sight-threatening complications that lead to poor vision and even blindness. 4 It has currently been indicated that no identified level of myopia can be considered safe concerning the ocular complications associated with it. 4 Thus, the concern of slowing myopia progression and ultimately preventing its onset has grown significantly. This has generated significant research interest in developing effective methods to slow the progression of myopia and/or delay its onset. Several clinical trials have demonstrated the beneficial effects of various myopia control strategies, some of which have been adopted in mainstream clinical practice. This review provides an overview of established therapeutic approaches and evolving methods for controlling myopia onset and progression.
Search strategy and study selection
A comprehensive literature search was conducted across multiple databases, including MEDLINE, PubMed, EMBASE and Web of Science. The search terms included “Peripheral Defocus,” “Myopia,” “Myopia Progression,” “Myopia Incidence,” “Myopia Management,” “Myopia Control,” “Atropine,” “Low Concentration Atropine,” “Low Dose Atropine,” “Repeated Low-Level Red Light,” “Low-Intensity Red Light,” “Red Light,” “Bifocal,” “Progressive Addition Lenses,” “Orthokeratology,” “Contact Lenses,” “Spectacle Lenses,” “Levodopa,” “Outdoor time,” “Outdoor,” “Combination,” and “Combined,” in varying combinations. The search was defined using the Boolean operators “AND” and “OR”, as well as truncations. Published studies were considered eligible if they met the following criteria: (1) clinical trials, (2) included children, aged 17 years or younger, (3) reported axial length elongation and/or change in spherical equivalent refraction and (4) reported treatment with red light, atropine, optical interventions or outdoor time.
Increased time outdoors
Earlier research found that increased participation in weekly sports and outdoor activities was associated with a lower risk of developing myopia. 5 Later, the protective role of outdoor time against myopia came to prominence in the Sydney Myopia Study. 6 Epidemiological studies spanning diverse geographical locations have subsequently supported this conclusion. 7 Randomised clinical trials have also demonstrated the efficacy of outdoor time interventions in reducing myopia incidence. The Guangzhou school-based clinical trial showed a 23% relative reduction in incident myopia over 3 years with the addition of 40 min of outdoor time daily. 8 In the Recess Outside Classroom (ROC) studies in Taiwan, the relative reduction in the incidence of myopia was approximately 52% when children spent 80 min of outdoor time per day. 9 A later phase of the ROC studies found a lesser relative reduction in the incidence of myopia, but this was against a background of now coexisting mandated time to be spent outdoors in schools in Taiwan. 10 Limited evidence suggests outdoor time may slow myopia progression. Wu et al found a 30% reduction in myopia progression in children, 10 aligning with reports showing slower myopia progression during summer compared with winter. 11 12 Recent corroborating evidence indicated accelerated myopia progression during the COVID-19 lockdowns in China and Hong Kong, suggesting a correlation with reduced outdoor time when compared with the pre-COVID-19 and post-COVID-19 eras. 13 14
In clinical settings, incorporating clinical recommendations to increase outdoor time could be beneficial in managing myopia progression. Discussing the benefits of spending more time outdoors and providing specific recommendations to children can help reduce the risk of myopia development and progression. Outdoor time is a simple and cost-effective intervention although the optimal duration, pattern and frequency for myopia prevention is unclear. Current recommendations suggest at least 2 hours per day. Longer or more frequent outdoor sessions may be more effective, but UV protection measures are important considerations. For optimal outcomes, it is important to consider using outdoor time as a complement to other myopia control interventions. A recent study demonstrated that decreased time outdoors during COVID-19 confinements reduced the treatment efficacy of Defocus Incorporated Multiple Segment (DIMS) spectacle lens. 15 Clinicians have a crucial role in advocating for the relevant public health interventions for myopia and promoting best clinical practices. Practitioners should provide advice on lifestyle and the visual environment, especially to patients whose parents or siblings are myopic, as well as those with high-risk ancestry, 16 17 such as encouraging a minimum of 2 hours of daily outdoor time 6 18 and less than 2 hours per day of leisure near work. Increasing viewing distance and having frequent breaks during near work (with any material) should also be recommended. 19–21
Beyond the clinic, promoting outdoor time can serve as a policy-level intervention, especially in educational settings. This approach may include creating environments and implementing policies that encourage outdoor activities, such as incorporating outdoor tasks into school curricula and providing dedicated time for outdoor activities during recess periods. Locations such as Taiwan, Singapore and China have pioneered outdoor programmes. The Ministry of Education in Taiwan implemented the Tian-Tian 120 programme, advocating 120 min of daily outdoor activities, which reduced the national prevalence of poor visual acuity in primary school children from 49.4% in 2012 to 46.1% in 2015, reversing the increasing trend of 34.8%–50% observed from 2001 to 2011. 22 The implementation of Taiwan’s Sports and Health 150 programme, which promotes 150 min of exercise at school per week, is an additional valuable measure. 23 It is worth emphasising that it is the exposure to natural daylight during these activities that is key to preventing myopia, rather than exercise alone. 6 The Singapore programme ‘Kids for Nature’ aimed to provide an educational programme in National Parks as an incentive for primary school children and their parents to spend more time outdoors, however, compliance with the programme declined over 6 months. In China, the guidelines for myopia prevention prioritise the importance of spending time outdoors and emphasise the role of schools in facilitating this. The Chinese government’s ‘Double Reductions’ policy, aimed at reducing homework and after-school tutoring while increasing after-school care, indirectly facilitates more outdoor activities, providing an excellent opportunity for increased time outdoors. 24
The implementation of increased outdoor time in schools raises concerns about lowering educational standards. However, a cross-sectional analysis of data from the Shanghai Time Outside to Reduce Myopia (STORM) study suggests that increased outdoor time may play a positive role in increasing academic performance. 25 This observation finds support in the 2018 rankings of the Organisation for Economic Co-operation and Development Programme for International Student Assessment, which indicates that many European countries achieved reading performance levels similar to those of Hong Kong without a myopia epidemic. 26 The real challenge is that in a modern environment of digital education, children may be heavily used to a more indoor lifestyle and feel reluctant to disconnect from excessive use of their electronic devices, even when they are spending time outdoors. In addition, the myopia epidemic had already reached a critical stage before the widespread adoption of computers and smartphones. Therefore, simply reverting to using books as a solution is not effective. Instead, addressing the problem requires efforts to reduce overall near-work activities and promote increased time outdoors. The proposed mechanism, which suggests that increased retinal dopamine release due to brighter light outdoors slows the rate of axial elongation, has been supported by studies conducted on experimental myopia in animals. 27 Preliminary findings suggest that this mechanism may also extend to humans. 28
Pharmacological interventions
Low-concentration atropine.
Atropine, an antagonist to acetylcholine muscarinic receptors, was initially used for myopia control based on the hypothesis that excessive accommodation of the eye contributes to myopia. However, subsequent animal studies revealed that accommodation was not involved and that non-accommodative mechanisms contribute to the development of myopia. 29 30 Thus, the focus of research has shifted towards non-accommodative mechanisms. This has involved a search for the specific site of action of atropine through studies directed at the posterior segment of the eye as the location of action of the antimyopic effect of atropine. Evidence has been presented against the sclera as a site of action for muscarinic antagonist control of myopia, 31 32 leaving the retina as the most likely site per the available evidence. Atropine rapidly reversed the downregulation of retinal ZENK mRNA during form deprivation in chicks, resulting in elevated expression levels compared with control eyes, indicating a strong likelihood of a retinal site of action. 33 However, whether atropine acts on muscarinic receptors in controlling axial elongation is not clear. Other studies have suggested possible interactions with a non-muscarinic receptor in the retina, specifically ɣ-aminobutyric acid receptors, suggesting a potential off-target effect. 34
Clinical trials have shown that atropine is potentially effective in slowing myopia progression and axial elongation ( table 1 ).
- View inline
Summary of trials on atropine for myopia control
Earlier reports found that 1% atropine conferred significant axial elongation and myopia control effects but was associated with greater rebound and adverse events, limiting its clinical uptake. 35 36 This resulted in a transition towards using lower concentrations, and a significant advancement came with the use of 0.01% in the Atropine for the Treatment of Childhood Myopia (ATOM) 2 study, which showed that 0.01% atropine was the most effective over 5 years compared with 0.1% and 0.5%, with minimal side effects and rebound. 37 38 However, the absence of a placebo group in the ATOM 2 study sparked debates and controversies surrounding its findings. Subsequent placebo-controlled trials in Asia, 39–44 as well as in Europe, Australia and the USA yielded inconsistent efficacies of 0.01% atropine ( table 1 ). 45–48
The low-concentration atropine for myopia progression (LAMP) study introduced a placebo group and investigated 0.05%, 0.025% and 0.01% atropine eye-drops for myopia control and found a concentration-dependent effect. The findings indicated a satisfactory safety profile that persisted for 3 years. 39 49 50 Notably, younger children showed less treatment effectiveness, requiring the higher concentration of 0.05% atropine to achieve a similar reduction in myopia progression as older children using lower concentrations. 51 Additionally, it was evident that low-concentration atropine induced choroidal thickening along a concentration-dependent response. 52 The LAMP study suggests that 0.05% atropine optimally balances efficacy and safety. It is currently being used for myopia control in Hong Kong but has not gained a widespread clinical uptake in other regions of the world. In light of the limited efficacy of 0.01% atropine in American and European children in recent large randomised controlled trials (RCTs), 45–47 there have been suggestions to use higher concentrations of atropine, particularly when addressing axial length elongation in white children. 47 53 54 Shifting to the relatively high concentrations of 0.05% atropine will ensure timely implementation of an effective dose for myopic children, especially when they are younger and/or at greater risk of rapid progression, serious eye complications and vision loss.
Atropine eye-drops have also been explored in the prevention of myopia onset. An earlier prospective study by Fang et al found that 0.025% decreased the onset of myopia in premyopic children aged 6–12 years. 55 Subsequent findings by the LAMP2 clinical trial showed that nightly use of 0.05% atropine resulted in a 46.4% reduction in a 2-year cumulative myopia incidence in non-myopic children aged 4–9 years. 56 Results from a cross-over trial also revealed that 0.01% atropine reduced myopia incidence among children aged 6–12 years. 57 The long-term myopia prevention effect needs further validation. Additionally, it is unclear how early atropine treatment should be initiated. One significant challenge is identifying premyopes who could benefit from the delaying effect of atropine, particularly since they may not seek eye examinations until after myopia has already developed, highlighting the need for public health approaches, enhanced screening methods and prediction models. China has made significant progress in the process of screening for refractive errors in schools, and this is now being expanded nationwide. 24 58 This is expected to help address the issue of clinical referral of premyopes, who may also tend to be pseudomyopes. While atropine is promising for myopia prevention, the extent to which its need for intervention can be prevented with increased time outdoors has not been specifically studied. Thus, it is recommended to consider atropine alongside outdoor time when managing premyopic children. There have been expressions of concern regarding the sustained efficacy of atropine eye-drops and the possibility of rebound effects occurring following cessation of treatment. The rebound effect refers to the resurgence of a condition after the discontinuation of treatment, resulting in a more rapid progression in the treatment than in the control group and the loss of accumulated effect. The ATOM1 and ATOM2 studies, 36 37 and subsequently the LAMP study, 50 demonstrated a concentration-dependent rapid axial elongation and myopia progression following the cessation of treatment. While tapering off 1% atropine has been shown to reduce rebound, 59 it is currently unclear whether this is also the case for lower concentrations. The mechanisms underlying the rebound phenomenon are currently unknown.
Continuing efforts to enhance treatment outcomes for myopia have led researchers to investigate and develop new interventions. In recent investigations, levodopa has shown promise as a potential treatment option. In a clinical setting, it is common practice to combine levodopa with carbidopa to prevent its early conversion to dopamine before it reaches the desired tissue. This coadministration approach plays a vital role in enhancing treatment outcomes. 60 Preclinical research involving animal models has demonstrated that the application of levodopa/carbidopa in a topical form can effectively inhibit experimental form-deprivation myopia and that induced by optical manipulation. 61–63 This inhibitory effect shows a dose-dependent relationship, with higher concentrations of levodopa/carbidopa offering complete protection. 61 63 Additionally, these findings highlight a significant increase in treatment efficacy when using topical levodopa/carbidopa compared with levodopa alone. 62 Consistent with the safety findings in preclinical studies, the first RCT demonstrated that the daily application of an ophthalmic solution containing levodopa/carbidopa over a period of 1 month was well tolerated. This treatment did not cause any alterations in anterior surface integrity, visual function, ocular health or refraction/ocular biometry in healthy adult males. 60 These findings provide a foundation for future investigations into the effectiveness of levodopa/carbidopa eye-drops as a potential treatment for myopia in humans. The probable mechanism through which levodopa exerts its antimyopic effects is by stimulating the synthesis and release of dopamine within the retina. 62
Optical devices
Novel spectacle lenses.
It has long been known from animal studies that the wearing of a minus lens during development, which creates hyperopic defocus (ie, shifting the point of focus behind the retina), could induce compensatory axial elongation, resulting in a myopic eye. 64 This later generated the hypothesis of the peripheral hyperopic defocus theory, which was that early relative peripheral hyperopic defocus was the underlying cause of myopia. In support of this, Smith et al demonstrated in rhesus monkeys that laser ablation of the fovea did not hinder the development of myopia in response to optically induced relative hyperopic defocus or form deprivation. 65 The findings were corroborated by cross-sectional studies in humans, which reported greater relative peripheral hyperopia in myopic children compared with relative peripheral myopia in emmetropes and hyperopes. 66–68 A major hindrance to this argument was that it was unclear whether relative peripheral hyperopic defocus was the cause or the consequence of myopia. 67 However, subsequent evidence from longitudinal studies has shown that the emergence of relative peripheral hyperopic defocus is more a consequence of the development of myopia rather than a cause since it manifests simultaneously with the onset of myopia and does not precede it. 69–71 While these findings indicated that foveal visual signals are not essential for regulating axial length growth, they did not conclusively prove that central defocus has no impact on refractive error development, and this is what later informed the simultaneous competing defocus theory as a potential mechanism for defocus myopia control spectacle and contact lenses. 72–74 It involves achieving clear central vision while simultaneously inducing relative myopic defocus across a significant portion of the peripheral retina. The theory is based on the principle that in situations where competing defocus signals occur simultaneously, the least hyperopic or more myopic focal plane takes precedence and exerts a stronger influence on refractive development. Although there have been studies on simultaneous competing defocus, the basis of myopia control spectacle and contact lens designs stems more from research emphasising the importance of myopic defocus on its own rather than from competing defocus.
MyoVision lenses: reducing relative peripheral hyperopic defocus
The first trial on defocus spectacle lenses tested three highly aspherised designs intended to reduce relative peripheral hyperopic defocus. The lenses differed in the size of the central optic zone and the magnitude of the relative positive power in the periphery. 75 The only successful lens type, later named MyoVision lenses, significantly slowed myopia progression by 30% only in a subgroup of children aged 6–12 years with parental history of myopia but had no significant effect on axial length elongation. Later, a multicentre clinical trial in Japanese children with a family history of myopia found no significant difference in myopia progression between MyoVision lenses and mono-focal glasses, 76 indicating that the lenses had less useful clinical impact. This implies that reduction of relative peripheral hyperopia may be insufficient to control myopia progression.
Multiple segments spectacle lenses: inducing myopic defocus in the mid-periphery of the retina
The DIMS spectacle lens was designed following the success of the Defocus Incorporated Soft Contact (DISC) lens that imposed myopic defocus across the retina. 77 The spectacle lenses, unlike DISC, were designed to have a central zone (diameter 9 mm) of myopic refractive correction giving clear vision and a surrounding zone of lenslets that create myopic defocus across the mid-periphery of the retina. 78 In a 2-year RCT, Chinese children aged 8–13 years who wore DIMS spectacle lenses had myopia progression retarded by 52% and axial length elongation by 62%. 78 Approximately 21% of the children who wore the DIMS spectacle lenses had no myopia progression over the 2-year period. Subsequent findings of the study demonstrated that DIMS lenses offered sustained efficacy over the course of 6 years without any negative side effects. 79 Clearly, the DIMS spectacle lenses are an effective and safe approach to managing progressive myopia in children.
Another version of a spectacle lens that uses multiple segments of myopic defocus has been developed by Essilor 80 and uses multiple concentric rings of lenslets of varying myopic defocus, described as aspherical lenslets. 81 These spectacle lenses also feature a central optical zone intended for correcting distance refractive error. The aspherical lenslets have been designed to deviate light in a manner that creates a volume of myopic defocus. 80 Thus, the size of the functional zone responsible for generating mid-peripheral myopic defocus is greater but does not result in a better efficacy than the DIMS lens. In a 2-year trial, it was found that lenses with a highly aspherical lenslets target (HALT) were more effective than single vision lenses in slowing myopia progression in Chinese children aged 8–13 years (reduced myopia progression by 55% and axial elongation by 51%), over a period of 2 years. 80 Additionally, the concentric aspherical design demonstrated a dose-dependent efficacy concerning both the degree of asphericity of the lenslets and the duration of lens wear. 80 82 In the third-year report, children who continued to wear the HAL demonstrated less axial elongation and myopia progression compared with those who wore single-vision lenses and are currently being followed for two more years. 83 Efforts can be directed towards refining the HALT design by increasing asphericity of the lenslets while maintaining wearer comfort. Finding this delicate balance could be instrumental in further improving the efficacy of HALT lenses. These lenses are marketed as the Stellest myopia control glasses.
Diffusion optics spectacle lenses
Contrast modulation is a novel mechanism of action employed in the Diffusion Optics Technology (DOT) spectacle lenses. 84 The lens is designed to control myopia by slightly reducing contrast at the retina by softly scattering incoming light to the eye. 84 The design is based on the principle that low contrast visual experience, like that from a natural outdoor environment, weakly stimulates the visual system and elicits low level, more natural bipolar cell activity that does not appear to disrupt normal eye growth, 85 whereas elevated contrast signalling in the retina, whether from genetic predisposition 84 86 or the modern urban visual environment, may overstimulate bipolar cells leading to overstimulation of axial elongation and myopia progression. 85 The DOT lens consists of a central clear aperture surrounded by a pattern of numerous micro-dot scattering centres spanning the treatment zone of the lens from edge to edge, each dot gently dispersing light to slightly lower artificial contrast to mimic more natural contrast.
In a 2-year clinical trial, DOT lenses were more effective compared with control lenses, delaying myopia progression by 59% and axial elongation by 38% over 2 years. 87 After 4 years, the lenses remained safe and more effective compared with standard single-vision control lenses. 88
Bifocal and progressive addition spectacle lenses
Bifocals and progressive addition lenses were initially considered for myopia management because they reduce accommodative lag and near-point esophoria, which have been associated with myopia progression. However, available studies using bifocals indicate either a slight effect or effectiveness limited to a specific subset of children with accommodative lag or near esophoria. 89 90 Other studies also suggest that progressive addition lenses have limited efficacy in controlling myopia, again often restricted to children with accommodative lags or near esophoria. 91–93 Thus, bifocals and progressive addition lenses cannot be considered universally effective interventions and do not achieve meaningful clinical benefits for the majority of myopic children.
To conclude, the reported outcomes suggest that DIMS, HALT and DOT lenses are effective and demonstrate significant clinical benefits. Myopia control spectacle lenses provide the added benefits of being safe and less invasive ( table 2 ). The lenses are easy to integrate into daily wear, requiring minimal adjustments to visual habits. However, some of these spectacle-based interventions may reduce high-contrast visual acuity and contrast sensitivity at higher spatial frequencies when viewed off-axis through the myopia control elements ( table 2 ). 81 94 95 There is no evidence to suggest that any of these side effects influence compliance with the lenses.
Efficacy, benefits and limitations of myopia control Interventions
Contact lenses
Orthokeratology lenses.
Orthokeratology (Ortho-K) uses overnight gas-permeable lenses to reshape the cornea for myopia correction. On morning removal, the flattened central cornea improves distance vision eliminating the necessity for glasses or contact lenses. This is especially convenient for individuals who prefer not to wear optical corrections during waking hours or engage in sports or activities where glasses or contact lenses may hinder their performance. In 2005, a pilot study suggested that Ortho-K might also reduce myopia progression. 96 In searching for an explanation of this effect, reports have suggested that Ortho-K not only reshapes the central cornea but also induces relative peripheral myopic defocus 97 through changes in spherical aberration. 98 Studies in Asia have reported significant axial length control efficacies of 36%–46% over 2 years. 96 99–101 This provides an interesting parallel to the findings of similar 2-year studies conducted in Spain (32%), Denmark (59%) and the USA (55%). 102–104 Earlier reports suggest that the efficacy of Ortho-K is particularly notable during the first and second years of treatment, especially when it is initiated at an early age of 6–8 years. 105 106 However, successful Ortho-K fitting requires advanced imaging and practitioner expertise, leading to longer clinic visits and added costs. In addition, even successful treatments may increase corneal irregular astigmatism and higher-order aberrations, sometimes reducing contrast sensitivity. 107 108 Moreover, sleeping in contact lenses creates an environment for the development of cases such as Acanthamoeba and Pseudomonas keratitis which can result in permanent scarring of the cornea and, in severe cases, vision loss. 109
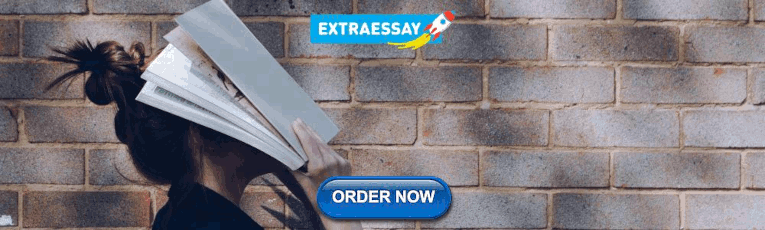
Multifocal soft contact lenses
Similar to findings from novel spectacle lenses, there is a consensus that soft contact lenses with a central distance zone and peripheral positive power can slow myopia progression. In this section, we explore two types of soft contact lenses for myopia control: progressive peripheral add (multifocal) contact lenses and concentric ring (bifocal) soft contact lenses ( table 3 ).
Summary of results of trials evaluating the myopia control effects of myopia control soft contact lenses
Both designs have a central zone for myopic correction. Peripheral addition lenses gradually adjust defocus from the centre to the periphery 110 while concentric ring bifocal lenses alternate between distance correction and plus power addition. 77
Progressive peripheral add soft contact lenses: multifocal
Based on the theory of reducing relative peripheral hyperopia to control myopia and using similar principles as the MyoVision spectacle lenses, contact lenses were developed that increased the level of reduction in relative peripheral hyperopia and increased the size of the treatment zone, moving it closer to the visual axis than had been designed for the spectacles. The contact lenses had a maximum add power of +2.00 D, and over 12 months, reduced myopia progression and axial length elongation by 34% and 33%, respectively, 110 but further longitudinal outcomes were not reported. Other studies employing progressive peripheral add power reaching +2.00 D have reported 2-year axial length and myopia control efficacies of 27%–29% and 43%–50%, respectively. 111 112 The BLINK study reported comparable results for lenses with a maximum add power of+2.50 D, showing a 36% decrease in axial elongation and a 43% decrease in myopia progression over a 3-year period. 113 Increasing the added power to a maximum of +3.00 D, Raffa et al observed a 66.6% control of myopia progression and 63.2% reduction in axial elongation over a period of 18 months. 114 An effort was made to use contact lenses with positive spherical aberration to control myopia by correcting retinal hyperopic blur caused by negative spherical aberrations associated with accommodation. Although the lens achieved a 38.6% reduction in axial length elongation over the course of a year, it was deemed clinically unviable as this reduction did not lead to a significant effect on the progression of myopia. 115 Given that spherical aberrations are not the sole higher-order aberrations linked to myopia, 116 the outcomes were not unexpected.
Concentric ring bifocal soft contact lenses
In a 2-year clinical trial, the DISC which later led to the development of DIMS, exhibited 32.4% less axial elongation and a 25% reduction in myopia progression. 77 The DISC was never released for commercial availability. MiSight contact lenses are dual-focus soft contact lenses with a central correction zone surrounded by a series of treatment and correction zones producing 2.00 D of simultaneous myopic retinal defocus. These have demonstrated up to 52% decrease in axial elongation and a 59% reduction in myopia progression over 3 years. 117–119 Aller et al reported a 72% decrease in refraction and 80% reduction in axial elongation with multi-ring bifocal soft contact lenses (Acuvue Bifocal by Vistakon), but this was in children with eso fixation disparity. The Acuvue Bifocal has been discontinued. 120
Unlike the typical dual-focus or multifocal soft contact lens designs, the non-coaxial ring focus contact lens generates a ring focus that falls in front of the retina but off the line of sight, enabling a larger treatment zone and the incorporation of a higher add power while maintaining comparable visual performance. 121 To examine the balance between reducing myopia and improving visual quality, two experimental lenses were used: one designed to enhance efficacy (EE) and the other to enhance vision. Both lens types consist of two concentric, annular treatment zones of +7.00 D non-coaxial plus power with the EE design featuring an additional +10 D coaxial treatment zone for greater efficacy without compromising visual acuity, due to the dispersal of the light rays. The EE lens (commercialised as ACUVUE Abiliti TM ) produced the most significant effect with a mean difference in axial length elongation being 0.11 mm compared with the control.
Efficacy of the peripheral addition multifocal contact lenses seems to be dependent on the magnitude of the add power and zones of correction, with high-addition lenses yielding relatively better outcomes. A consensus on the optimal amount of defocus and the ideal zones to correct is required. The MiSight and ACUVUE Abiliti lenses demonstrate efficacy levels comparable to those reported for the simultaneous competing defocus spectacle lenses, probably because of the similar design principles. Myopia control with soft contact lenses can serve as viable alternatives for patients who are not indicated for or are unsuccessful in undergoing orthokeratology treatment or who may not wish to wear spectacles. Soft contact lenses, placed directly on the eye, may provide a broader peripheral view and less distortion than spectacles, benefiting activities such as sports and driving ( table 2 ). Nonetheless, soft contact lens wear is associated with the risk of corneal infiltrative events and requires strict adherence to treatment regimen and lens care. 122
Red light therapy
Another emerging method for myopia control is light exposure therapy. Animal experiments had suggested that long-wavelength (red) light irradiation could produce a hyperopic shift in infant and juvenile rhesus monkeys as well as in infant tree shrews. 123 124 This is the reverse of what would be expected in longitudinal chromatic aberration, which involves matching the focal plane of the dominant wavelength by increasing the speed of growth when the dominant wavelength is long and decreasing the speed of growth when the dominant wavelength is short. 123 125 More recent human clinical trials have shown that red light therapy (RLT) is effective in controlling myopia progression over 1 and 2 years. 126 127 At 2 years, a 75% reduction in both axial length elongation and myopia progression was found, following a treatment plan of 3 min per session, twice daily and with a minimum interval of 4 hours. Comparable results have been reported in trials from other centres at 6 months and at 1 year. 128–131 RLT has also yielded up to 54.1% uninterrupted treatment efficacy in preventing incident myopia after a year. 132 Of note, the prophylactic effect in children whose spherical equivalent refraction was close to −0.50 D was lower, as the intervention was introduced late, implying that early intervention with RLT could confer a higher efficacy.
While the mechanisms are not fully understood, it is recognised that increased choroidal thickness through increased retinal blood flow and metabolism contributes to a minor portion of these observed changes. 133 134 It is unclear whether red light interacts directly with the cones or if the critical mechanism is photobiomodulation, which involves the reduction of nitrite to nitrous oxide through cytochrome c oxidase in response to red light.
Available evidence of safety suggests red light is a low-risk therapy that causes no significant adverse events and that it has been used in amblyopia treatment in China for over a decade with no long-term negative effects reported regarding its usage. 126 129–131 In a recent clinical case report, a 12-year-old girl experienced a decrease in best-corrected visual acuity for a period of 2 weeks following 5 months of regular exposure to RLT for myopia control. 135 Fundus photographs showed darkening of the foveae, hypoautofluorescence of the maculae, as well as OCT examination revealing disruptions in the bilateral foveal ellipsoid zone and interdigitation zone. After 3 months without RLRL therapy, the bilateral outer retinal damage recovered, and the visual acuity improved. Despite acknowledging potential retinal phototoxicity sensitivity and the fact that this could be an atypical case or a suspected Stargardt disease, it is crucial to maintain ongoing attention and vigilance by thorough clinical assessments and closely monitoring both the visual acuity, colour sensitivity and retinal health of patients throughout the treatment with RLT.
The available device can be administered at home and requires the child to sit by the device and use it for the recommended duration. This will allow parents to actively monitor adherence and ensure that the child follows the treatment regimen ( table 2 ). However, due to the size and power requirements of the device, it is less portable compared with the other interventions. Adopting lightweight designs like spectacles will enhance convenience and ease of use, especially during long periods of travel away from home. Additional long-term studies are encouraged to clarify the mechanism and safety of RLT for myopia control.
Combined therapy
Combined treatments, targeting multiple pathways, are emerging as a more effective approach to myopia control than monotherapy. Recent studies indicate that combining 0.01% atropine with Ortho-K consistently outperforms either treatment alone in slowing axial length elongation, especially in younger children with shorter baseline axial length. 136–138 While the precise mechanisms are not fully understood, it is plausible that since the mechanisms appear to be different, their effects could be cumulative.
The efficacy of atropine combined with myopia control spectacles has been investigated in a few studies. Shih et al demonstrated a significant additive effect of atropine 0.5% when multifocal spectacle lenses were also worn but not with multifocal spectacle lenses alone. 139 Similarly, enhanced efficacy has been found in combining 0.01% atropine with DIMS spectacle lenses compared with DIMS spectacle lens or atropine monotherapy, in both Chinese children 140 and European subjects. 141 The mechanism underlying the additive effect of atropine and spectacle lenses is unclear; however, the improved efficacy may be attributed again to the differing mechanisms or to the use of atropine resulting in larger pupil diameter, leading to increased retinal illumination and positive spherical aberration, and thereby enhancing the myopic defocus effects of optical interventions. In the contrast, the Bifocal and Atropine in Myopia study found that combining 0.01% atropine and soft multifocal contact lenses (SMCLs; add: +2.50 D) did not significantly improve myopia control over the SMCLs alone. 142
Future studies may focus on higher concentrations of atropine, such as 0.05% atropine, combining with orthokeratology to effectively control myopia in individuals with high myopia, fast myopia progression or poor response to orthokeratology. It is worth indicating that the enhanced efficacy of the combined therapy has only been investigated in children with low to moderate myopia, and it is necessary to exercise caution when applying results to other situations.
Conclusions and future directions
There has been significant advancement in the development of myopia control methods over the past few years, with some methods demonstrating an acceptable balance between efficacy and safety. Specifically, 0.05% atropine, DIMS spectacle lenses, DOT lenses, lenses with HAL, RLT, concentric ring soft contact lenses and orthokeratology lenses demonstrate favourable outcomes. Among these, the myopia control spectacle lenses are the least invasive and safest although all have demonstrated tolerable safety profiles. The efficacy of peripheral addition soft contact lenses is inconsistent, and it is obvious that a consensus is needed on the optimal amount of defocus to incorporate and the ideal zones to correct. While outdoor time is well established for the prevention of myopia onset, atropine and RLT need further studies for clinical validation. Considering the rapid increase of myopia, promoting outdoor time for prevention, and reserving clinical interventions for refractory cases is essential for broader impact. It is important to recognise that there is an inherent growing of the eyeball and associated shift in refractive state that occurs in childhood, even in non-myopic children. Thus, the goal of myopia control interventions is not to completely halt these natural changes but to reduce the risk of developing high myopia and subsequent sight-threatening complications. Therefore, the level of myopia control that can be deemed effective and protective is when an intervention reduces the progression of myopia at a rate that can be projected to prevent the development of high myopia or myopia-associated ocular complications by the time myopia stabilises. This emphasises the need for individualised approaches to myopia management, considering the rate of myopia progression, ethnicity, age, severity of myopia and potential adverse effects.
Variations in ethnicity and age groups of participants have been major hindrances to extrapolating results and directly comparing the effectiveness of different treatment methods. This may make it challenging for eye care practitioners to confidently recommend the most effective intervention to their patients. There has not been widespread clinical uptake of some potentially efficacious interventions like red light and atropine 0.05% and this could be due to the predominantly Asian population of the participants who were enrolled in the trials. Future trials involving other races are warranted.
Finally, it should be noted that these therapies are directed at slowing excessive axial elongation of the eye, rather than affecting other optical components. While axial elongation is the predominant reason for the generation of myopia, it is worth noting that there are at least two other circumstances that can lead to myopic refractive errors, namely keratoconus and myopia of prematurity that is lenticular in origin. The use of the treatments to slow axial elongation is likely not to be appropriate in these cases, and therefore, preliminary ocular biometric measures and birth history must be considered carefully before commencement of these therapies. It is yet unclear how efficacious these treatments might be in cases of syndromic myopia and possibly familial high myopia, which are likely to be genetic in origin. Trials in children affected with these conditions have yet to be conducted and should be approached with the clear understanding that outcomes are unknown.
Ethics statements
Patient consent for publication.
Not applicable.
- Kam KW , et al
- Morgan IG ,
- Ohno-Matsui K ,
- Holden BA ,
- Fricke TR ,
- Wilson DA , et al
- Haarman AEG ,
- Enthoven CA ,
- Tideman JWL , et al
- Sinnott LT ,
- Mutti DO , et al
- Ip J , et al
- Sankaridurg P ,
- Naduvilath T , et al
- Zeng Y , et al
- Wu H-L , et al
- Lin K-K , et al
- Gwiazda J ,
- Manny R , et al
- Donovan L ,
- Ho A , et al
- Lin L , et al
- Tang WC , et al
- Tarczy-Hornoch K ,
- Cotter SA , et al
- Farbrother JE ,
- Owen MJ , et al
- Jiang W-J , et al
- Ramamurthy D ,
- Lin Chua SY ,
- Cheng Q , et al
- Huang P-C ,
- Hsiao Y-C ,
- Tsai C-Y , et al
- Chang L-C , et al
- Schaeffel F
- Gurlevik U ,
- McBrien NA ,
- Moghaddam HO ,
- Schaeffel F ,
- Wallman J , et al
- McCarthy CS ,
- Maleszka R , et al
- Barathi VA ,
- Chaurasia SS ,
- Poidinger M , et al
- Balakrishnan V ,
- Chan Y-H , et al
- Koh ALT , et al
- Wen L , et al
- Tang SM , et al
- An W , et al
- Gupta V , et al
- Ng ALK , et al
- Jain A , et al
- Hiraoka T ,
- Fujikado T , et al
- Schulman E ,
- Flitcroft I , et al
- Loughman J ,
- Kobia-Acquah E ,
- Lingham G , et al
- Chandler DL , et al
- Lingham G ,
- Blaszkowska M , et al
- Zhang X , et al
- Zhang Y , et al
- Lee J , et al
- Phillips JR
- Walline JJ ,
- Berntsen DA
- Yu HJ , et al
- Yu S , et al
- Guo L , et al
- Thomson K ,
- Karouta C ,
- Sabeti F , et al
- Karouta C , et al
- Kelly T , et al
- Morgan I , et al
- Glasser A ,
- Ramamirtham R ,
- Qiao-Grider Y , et al
- Sholtz RI ,
- Friedman NE , et al
- Gazzard G , et al
- Donovan L , et al
- Smith Iii EL ,
- Arumugam B ,
- Hung L-F , et al
- To CH , et al
- Guggenheim JA , et al
- Varnas S , et al
- Hiraoka T , et al
- Tse DY-Y , et al
- Zhang HY , et al
- Li X , et al
- Li Y , et al
- Huang Y , et al
- Yin Z , et al
- Young G , et al
- SightGlass Vision
- Wagner-Schuman M ,
- Rowlan JS , et al
- Neitz M , et al
- Chalberg T ,
- Laughton D ,
- Hill J , et al
- Drobe B , et al
- Correction of Myopia Evaluation Trial 2 Study Group for the Pediatric Eye Disease Investigator Group
- Gwiazda JE ,
- Norton TT , et al
- Edwards MH ,
- Lam CS-Y , et al
- Bullimore MA ,
- Ritchey ER ,
- Shah S , et al
- Cheung SW ,
- Swarbrick H
- Atchison DA
- Cheung JKW ,
- Wong GTK , et al
- Jakobsen TM ,
- Santodomingo-Rubido J ,
- Villa-Collar C ,
- Gilmartin B , et al
- VanderVeen DK ,
- Kraker RT ,
- Pineles SL , et al
- Okamoto C ,
- Ishii Y , et al
- Okamoto F ,
- Kaji Y , et al
- Li GKH , et al
- Smith E , et al
- Greiner KL ,
- McVey ME , et al
- Morales H ,
- Armengol J , et al
- Walker MK ,
- Allinjawi K , Sharanjeet-Kaur , et al
- Chehab K , et al
- Okamoto F , et al
- Chamberlain P ,
- Peixoto-de-Matos SC ,
- Logan NS , et al
- Anstice NS ,
- Ruiz-Pomeda A ,
- Pérez-Sánchez B ,
- Valls I , et al
- Wildsoet CF
- Chalmers RL ,
- Mitchell GL , et al
- Arumugam B , et al
- Jiang Y , et al
- Tan X , et al
- Liao H , et al
- Xu H , et al
- Liao Y , et al
- Ma D-L , et al
- Zhu Z , et al
- Liang S-T , et al
- Guo J , et al
- Zhou J , et al
- Kinoshita N ,
- Hamada N , et al
- Cheng GP , et al
- Chen CJ , et al
- He T , et al
- Schiavetti I , et al
- Jones-Jordan LA , et al
- Cheung Y-B , et al
- Chou AC , et al
- Stapleton F ,
- Wei L , et al
- Lyu Y , et al
XJZ and EZ are joint first authors.
X @JasonYam7
XJZ and EZ contributed equally.
Contributors EZ and XZ contributed equally. XZ conducted the literature search, article selection, data extraction, preparation of tables and wrote the main manuscript. EZ conducted the literature search, article selection, data extraction, preparation of tables and wrote the main manuscript. FYT carried out the data extraction, prepared the tables and edited the main manuscript. KWK and ANF critically revised the main manuscript. CCT carried out data interpretation and critically revised the main manuscript. LJC carried out data interpretation and critically revised the main manuscript. C-PP carried out data interpretation and critically revised the main manuscript. JCY designed the study, carried out data extraction and interpretation, prepared the tables and critically revised the manuscript.
Funding The authors declare that they have no known competing financial interests or personal relationships that could have appeared to influence the work reported in this paper
Competing interests None declared.
Provenance and peer review Commissioned; externally peer reviewed.
Read the full text or download the PDF:
Effect of Repeated Low-Level Red-Light Therapy for Myopia Control in Children: A Multicenter Randomized Controlled Trial
Affiliations.
- 1 State Key Laboratory of Ophthalmology, Zhongshan Ophthalmic Center, Sun Yat-sen University, Guangzhou, China.
- 2 Hunan Key Laboratory of Ophthalmology, Eye Center of Xiangya Hospital, Central South University, Changsha, Hunan, China.
- 3 Department of Ophthalmology, Affiliated Foshan Hospital, Southern Medical University, Foshan, 528000, China.
- 4 Department of Ophthalmology, Shenzhen Children's Hospital, Shenzhen, Guangdong, China.
- 5 State Key Laboratory of Ophthalmology, Zhongshan Ophthalmic Center, Sun Yat-sen University, Guangzhou, China. Electronic address: [email protected].
- 6 Research School of Biology, Australian National University, Canberra, Australia.
- 7 State Key Laboratory of Ophthalmology, Zhongshan Ophthalmic Center, Sun Yat-sen University, Guangzhou, China; Centre for Eye Research Australia, Royal Victorian Eye and Ear Hospital, Melbourne, Australia; Ophthalmology, Department of Surgery, University of Melbourne, Melbourne, Australia. Electronic address: [email protected].
- PMID: 34863776
- DOI: 10.1016/j.ophtha.2021.11.023
Purpose: To assess the efficacy and safety of repeated low-level red-light (RLRL) therapy in myopia control in children.
Design: Multicenter, randomized, parallel-group, single-blind clinical trial.
Participants: Two hundred sixty-four eligible children 8 to 13 years of age with myopia of cycloplegic spherical equivalent refraction (SER) of -1.00 to -5.00 diopters (D), astigmatism of 2.50 D or less, anisometropia of 1.50 D or less, and best-corrected visual acuity (BCVA) of 0.0 logarithm of the minimum angle of resolution or more were enrolled in July and August 2019. Follow-up was completed in September 2020.
Methods: Children were assigned randomly to the intervention group (RLRL treatment plus single-vision spectacle [SVS]) and the control group (SVS). The RLRL treatment was provided by a desktop light therapy device that emits red light of 650-nm wavelength at an illuminance level of approximately 1600 lux and a power of 0.29 mW for a 4-mm pupil (class I classification) and was administered at home under supervision of parents for 3 minutes per session, twice daily with a minimum interval of 4 hours, 5 days per week.
Main outcome measures: The primary outcome and a key secondary outcome were changes in axial length and SER measured at baseline and the 1-, 3-, 6-, and 12-month follow-up visits. Participants who had at least 1 postrandomization follow-up visit were analyzed for treatment efficacy based on a longitudinal mixed model.
Results: Among 264 randomized participants, 246 children (93.2%) were included in the analysis (117 in the RLRL group and 129 in the SVS group). Adjusted 12-month axial elongation and SER progression were 0.13 mm (95% confidence interval [CI], 0.09-0.17mm) and -0.20 D (95% CI, -0.29 to -0.11D) for RLRL treatment and 0.38 mm (95% CI, 0.34-0.42 mm) and -0.79 D (95% CI, -0.88 to -0.69 D) for SVS treatment. The differences in axial elongation and SER progression were 0.26 mm (95% CI, 0.20-0.31 mm) and -0.59D (95% CI, -0.72 to -0.46 D) between the RLRL and SVS groups. No severe adverse events (sudden vision loss ≥2 lines or scotoma), functional visual loss indicated by BCVA, or structural damage seen on OCT scans were observed.
Conclusions: Repeated low-level red-light therapy is a promising alternative treatment for myopia control in children with good user acceptability and no documented functional or structural damage.
Keywords: Axial length; Myopia control; Randomized clinical trial; Repeated low-level red-light therapy; Spherical equivalent refraction.
Copyright © 2021 American Academy of Ophthalmology. Published by Elsevier Inc. All rights reserved.
Publication types
- Multicenter Study
- Randomized Controlled Trial
- Research Support, Non-U.S. Gov't
- Disease Progression
- Phototherapy
- Refraction, Ocular
- Single-Blind Method
2023-4 IMI Myopia Attitudes and Practices Survey
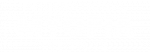
IMI 2023 White Papers Introduction
Imi clinical summary, taskforce chair.
- Dr Nina Tahhan
Taskforce Members
- James S. Wolffsohn
- Padmaja Sankaridurg
- Jost B. Jonas
- Mark A. Bullimore
- Ian Flitcroft
- Lisa A. Ostrin
- Christine Wildsoet
- Serge Resnikoff
Available Languages
This IMI Clinical Summary is also available for download in the following languages:
IMI white papers are published in response to the growing need for consensus and clinical management guidance on the ever growing, and sometimes conflicting, evidence base around myopia development and management. Consolidation, consensus, and updates on all the latest evidence in the form of these white papers is an important resource for practicing clinicians who may not have the time and resources available to sift through the ever evolving and growing body of evidence to understand how the most recent findings translate to clinical practice and how to implement the most appropriate and effective treatment strategies. All IMI articles and associated infographics that are freely available serve as tools to help with this process. By highlighting gaps in our current knowledge, they also provide a guide for ongoing and future research.
This third series of white papers published in 2023 highlights key areas of myopia research and management which have been gaining interest. These include:
- young adults
- pediatric (infant and pre-school children less than 5 years of age)
- Emerging evidence for roles of the choroid in both myopia development and myopia control. The growing evidence in this field warrants further attention, particularly for clinicians who may be grappling to understand how research findings might translate to clinical practice.
- A thorough characterization of non-pathological ocular changes in myopia which may help researchers to further elucidate the mechanism of axial elongation and better understand associated secondary pathologies
In addition, a report on the results of an international survey of practitioners on myopia management attitudes and strategies in clinical practice is included. This paper reflects on how practices and attitudes regarding myopia management may have changed over the past decade based on other similar, previously published survey results. The latest results indicate that single vision spectacles and contact lenses are still the most prescribed methods of correction, although clinical activities related to myopia management, including the prescription of myopia control devices and therapies, appear to be increasing. More needs to be done to establish myopia control as the standard of care for progressive myopia around the world.
To help stakeholders keep up to date with this fast-moving field, new findings across some of the key topics in myopia research since the 2019 digest have been reviewed by experts and summarized as the IMI 2023 digest.
By 2050, it is predicted that almost half of the global population will be myopic, with 10% at levels worse than −5.00 diopters and hence at greater risk of sight-threatening complications and visual impairment. Every diopter matters and hence every clinician should be supported and encouraged to introduce evidence-based myopia management to improve the quality of life and well-being of their patients, their families, communities, and the broader society. We commend all those who are striving to make this change and thank all those who have contributed to these efforts. We also invite all who are willing and interested to join the IMI in these efforts.
ACKNOWLEDGMENTS
A full list of the IMI taskforce members and the complete IMI white papers can be found at myopiainstitute.org. The publication and translation costs of the clinical summary was supported by donations from BHVI, ZEISS, EssilorLuxottica, CooperVision, Alcon, HOYA, Théa, and Oculus.
Nina Tahhan, James S. Wolffsohn, Padmaja Sankaridurg, Jost B. Jonas, Mark A. Bullimore, Ian Flitcroft, Lisa A. Ostrin, Christine Wildsoet, Serge Resnikoff; Editorial: International Myopia Institute White Paper Series 2023. Invest. Ophthalmol. Vis. Sci. 2023;64(6):1. doi: https://doi.org/10.1167/iovs.64.6.1.
CORRESPONDENCE
Brien Holden Vision Institute Ltd Level 4, North Wing, Rupert Myers Building, Gate 14 Barker Street, University of New South Wales, UNSW NSW 2052 [email protected]
IMI White paper
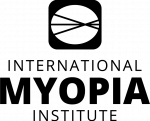
- Privacy Policy
- Terms & Conditions
Nina Tahhan, James S. Wolffsohn, Padmaja Sankaridurg, Jost B. Jonas, Mark A. Bullimore, Ian Flitcroft, Lisa A. Ostrin, Christine Wildsoet, Serge Resnikoff; Editorial: International Myopia Institute White Paper Series 2023. Invest. Ophthalmol. Vis. Sci. 2023;64(6):1. doi: https://doi.org/10.1167/iovs.64.6.1 .

Innovative 3D printing could revolutionize treatment for cataracts and other eye conditions
University of East Anglia researchers have made a significant breakthrough in ocular device technology with the introduction of a novel resin for 3D printing intraocular devices. This innovation has potential to enhance the manufacture of eye implants universally used in cataract and refractive surgeries.
An artificial intraocular lens (IOL) is primarily required for people with cataracts, a condition where the eye's natural lens becomes cloudy, obscuring vision.
They can also be also used to correct refractive errors such as myopia (nearsightedness), hyperopia (farsightedness) and presbyopia (when eyes gradually lose the ability to see things clearly up close, as a normal part of aging).
Lead author Dr Aram Saeed, Associate Professor in Healthcare Technologies at UEA's School of Pharmacy, said: "For the first time, we have developed a resin that can be used to print ocular devices directly.
"While still in the early stages, the ability to 3D print these lenses could significantly enhance eye care for patients by offering unprecedented levels of customisation and design precision, potentially leading to better clinical outcomes."
Historically, IOLs have been made from a variety of materials, including glass and silicone, although more recently the industry has significantly evolved to predominantly use acrylic materials.
Currently hydrophilic and hydrophobic acrylic are the most commonly used materials due to their excellent optical clarity, flexibility, biocompatibility with the body and for their stability and safety within the eye.
Current methods of making IOLs use lathing and moulding techniques. While these methods offer the production of well-engineered and high-optical quality devices, they also come with inherent limitations, particularly in terms of design complexity and customisation.
Dr Aram Saeed said: "3D printing could significantly enhance the production of ocular devices, not only improving speed and precision in manufacturing but also enabling greater complexity and customisation in design.
"Our proof-of-concept paper is the first in a series that will detail our developments in this area and set the stage for transforming eye care practices globally.
"Our work combines material science with healthcare technology and requires extensive know-how in developing these types of ocular devices.
"As we continue to publish our findings and share our advancements, we aim to be at the forefront of the industry, working with industrial partners and researchers worldwide to refine and enhance the technology."
Although still in the early stages of development, the innovation could potentially have several advantages:
Tailored Lenses : 3D printing could create lenses customised to each patient's eye shape and vision needs, potentially improving vision correction and comfort.
Faster Production : Compared to traditional methods, 3D printing has the potential to enable quicker design, testing, and manufacturing of lenses. This speed could reduce the time between diagnosis and surgery, providing faster care to patients.
Complex Designs : 3D printing makes it possible to create intricate lens shapes that were previously difficult to manufacture. These designs could better address a wider range of vision problems.
Cost Reduction : By using 3D printing, the production cost of custom or high-quality lenses may decrease, making them more affordable for more patients, particularly in economically disadvantaged regions. This could lead to better overall public health outcomes.
Compatibility with Imaging : The researchers hope that combining 3D printing with advanced imaging technologies in the future could help produce lenses that fit individual patients' eyes optimally, reducing the need for adjustments or complications after surgery.
Material Innovation : 3D printing allows for the development of new materials with improved optical performance. This could result in lenses that not only correct vision but also enhance it.
The study found that the 3D printed lenses have good optical clarity, can be folded, and implanted into a human capsular bag.
Co-author Michael Wormstone, Emeritus Professor at UEA's School of Biological Sciences, said: "If successful in further developments, this new technology could transform the industry by enabling portable manufacturing solutions, especially beneficial in remote and economically disadvantaged areas.
"It also has the potential to support the production of premium, customised lenses that could enhance surgical outcomes in more advanced healthcare settings."
The team's efforts have been recognised with the awarding of a United States patent, assigned to UEA Enterprise Limited, a business entity of the university focused on fostering innovation and commercialising research.
The UEA researchers continue to work closely with industry partners to refine the technology.
For example, further work has been underway to ensure the process works accurately on a larger scale and to increase the printing resolution to improve the dimensional accuracy.
It is hoped that clinical trials could start in the next few years.
Dr Saeed and Prof Wormstone have a strong partnership with the ophthalmology department at Norwich and Norfolk University Hospital (NNUH), which brings valuable clinical insights and visionary approaches to their work, with both UEA and the hospital members of the pioneering Norwich Research Park.
Mr Anas Injarie, a leading consultant ophthalmologist at NNUH with more than 20 years of experience, said: "This innovation has the potential to enable the production of lenses that match patient specifications in design and optical performance.
"For premium markets, it represents an exciting possibility to provide tailored treatments that could enhance patient satisfaction and surgical success."
The research was funded by the University of East Anglia through the Innovation Development Fund and Proof-Of-Concept grants; the Humane Research Trust; and the Engineering and Physical Sciences Research Council (EPSRC).
Further funding was provided by UEA's Impact Acceleration Account (IAA) funding from the Medical Research Council (MRC).
'Stereolithographic Rapid Prototyping of Clear, Foldable, Non-refractive Intraocular Lens Designs: A Proof-of-Concept Study' is published in the journal Current Eye Research .
- Today's Healthcare
- Patient Education and Counseling
- Diseases and Conditions
- 3-D Printing
- Medical Technology
- Engineering and Construction
- Contact lens
- Dominant eye in vision
- Refractive surgery
- Eye examination
- Visual acuity
- Formaldehyde
Story Source:
Materials provided by University of East Anglia . Note: Content may be edited for style and length.
Journal Reference :
- Veronica Hidalgo-Alvarez, Noelia D. Falcon, Julie Eldred, Michael Wormstone, Aram Saeed. Stereolithographic Rapid Prototyping of Clear, Foldable, Non-Refractive Intraocular Lens Designs: A Proof-of-Concept Study . Current Eye Research , 2024; 1 DOI: 10.1080/02713683.2024.2344164
Cite This Page :
Explore More
- Future Climate Impacts Put Whale Diet at Risk
- Charge Your Laptop in a Minute?
- Caterpillars Detect Predators by Electricity
- 'Electronic Spider Silk' Printed On Human Skin
- Engineered Surfaces Made to Shed Heat
- Innovative Material for Sustainable Building
- Human Brain: New Gene Transcripts
- Epstein-Barr Virus and Resulting Diseases
- Origins of the Proton's Spin
- Symbiotic Bacteria Communicate With Plants
Trending Topics
Strange & offbeat.

An official website of the United States government
The .gov means it’s official. Federal government websites often end in .gov or .mil. Before sharing sensitive information, make sure you’re on a federal government site.
The site is secure. The https:// ensures that you are connecting to the official website and that any information you provide is encrypted and transmitted securely.
- Publications
- Account settings
Preview improvements coming to the PMC website in October 2024. Learn More or Try it out now .
- Advanced Search
- Journal List
- HHS Author Manuscripts

An evidence-based update on myopia and interventions to retard its progression
Seo-wei leo.
a National Healthcare Group Eye Institute, Singapore
b Kandang Kerbau Women’s and Children’s Hospital, Singapore
Terri L. Young
c Eye Center, Department of Ophthalmology, Duke University, Durham, North Carolina
d Duke–National University of Singapore Graduate Medical School, Singapore
Associated Data
Myopia is the most common human eye disorder. With its increasing prevalence and earlier age-of-onset in recent birth cohorts, myopia now affects almost 33% of adult individuals in the United States, and epidemic proportions of 85% to 90% adult individuals in Asian cities. Unlike children in Western populations, where the prevalence of myopia is very low (less than 5%), Asian children have prevalences as high as 29% in 7-year-olds. In addition to the direct economic and social burdens of myopia, associated ocular complications may lead to substantial vision loss. This workshop summarizes the current literature regarding myopia epidemiology, genetics, animal model studies, risk factors, and clinical treatments. Published treatment strategies to retard the progression of myopia in children, such as pharmacologic agents, progressive addition lenses, neural adaptation programs are outlined.
Myopia, or near-sightedness, is the state of refraction in which parallel rays of light are brought to focus in front of the retina of a resting eye. 1 It is measured by the spherical power in diopters of the diverging lens needed to focus light onto the retina, which can be expressed as the spherical equivalent (SE), that is, sphere + half negative cylinder. Most commonly used definitions of myopia in epidemiologic studies include SE of at least −0.50D, −0.75D, and −1.0D. 2 Myopia is the most common human eye disorder in the world, affecting 85% to 90% of young adults in some Asian countries such as Singapore and Taiwan, 3 , 4 and between 25% and 50% of older adults in the United States and Europe. 5 – 7 Epidemiological studies in Western populations have collectively shown the prevalence of myopia to be low (<5%) in children aged 8 years or younger. 8 – 14 However, studies in Asian children suggest a significantly higher prevalence of myopia, affecting 9% to 15% of preschool children 15 , 16 and 29% of primary school children in Singapore. 17 A study of 10,000 Taiwanese school children found that the prevalence of myopia was 6% in 6-year-olds, with the prevalence increasing to more than 70% by age of 15 years. 18
With its increasing prevalence and earlier age of onset in recent birth cohorts, myopia now affects 33% of adults in the United States. Between 1999 and 2004, the prevalence of myopia was two-thirds higher than it was between 1971–1972. 19 The National Health and Nutrition Examination Survey (NHANES) also showed a higher prevalence in women (39.9%) than in men (32.6%), in younger than older persons, and in whites (35.2%) than African Americans (28.6%) or Mexican Americans (25.1%). 19
Myopia is a significant global public health concern. 19 Along with cataract, macular degeneration, infectious disease, and vitamin A deficiency, myopia is one of the most important causes of visual impairment worldwide. 20 , 21 Severe or high-grade myopia is a leading cause of blindness because of its associated ocular comorbidities of retinal detachment, macular choroidal degeneration, premature cataract, and glaucoma. 22 – 27 The yearly incidence of retinal detachments had been estimated as 0.015% in patients with less than 4.74 D myopia and increases to 0.07% in patients with myopia greater than or equal to 5 D and 3.2% in patients with myopia greater than or equal to 6 D. 22 , 23 Myopes also have increased risks of developing macular choroidal neovascularization, ranging from two times for patients with 1 D to 2 D of myopia, four times with 3 D to 4 D of myopia, and nine times for −5 D to 6 D. 24 – 26 The Blue Mountains Eye Study showed that glaucoma was present in 4.2% of eyes with low myopia and 4.4% of eyes with moderate to high myopia, compared to nonmyopic eyes. 27
Ample evidence supports heritability of the nonsyndromic forms of this condition, especially for high-grade myopia commonly referred to as myopic spherical refractive power of 5 D to 6 D or higher. 28
Epidemiology
Recent epidemiological data has identified outdoor activity as a key environmental determinant of myopia. In both Singaporean and Australian children, total time spent outdoors was associated with less myopic refraction, independent of indoor activity, reading, and engagement in sports. 29 , 30 A comparative study of Chinese children in Singapore and Sydney also revealed a protective effect of outdoor activity. 31 , 32
Previous reports of rural–urban differences in myopia prevalence have also been confirmed, with inner-city urban areas having higher odds of myopia than outer suburban areas. This data suggested that small to moderate environmental differences may affect myopia development, even within a common predominantly urban environment. 33
Genetics of Ocular Refractive Components
Refraction is determined by coordinated contributions of ocular biometric components such as axial length (AL), anterior chamber depth (ACD), corneal curvature (keratometry readings in diopters), and lens thickness. The inverse relationship of AL and ACD to refraction is well documented (the longer the eye, the more myopic the refractive error). Myopes have longer axial lengths, deeper vitreous chambers, thinner lenses, and flatter corneas. 34 – 36 In the vast majority of cases, the structural cause of myopia is an excessive axial length of the eye, or more specifically, the vitreous chamber depth. AL is estimated to be the greatest determinant of refractive error; heritability estimates for AL range from 40% to 94%, and most recently were reported to be 81% in a whole genome twin study in Australia. 37 This study was the first to identify a locus implicated in ocular axial length, on chromosome 5q, and it identified additional regions with suggestive multipoint logarithm of the odds (LOD) ratios on chromosomes 6, 10, and 14 linked to axial length. 37
Twin Studies
Twin studies provide the strongest conclusive evidence that myopia is inherited, as background contributions are diminished. Many studies have noted an increased concordance of refractive error as well as refractive components (AL, corneal curvature, lens power) in monozygotic twins compared to dizygotic twins. Most recently, Dirani and colleagues 38 reported the first evidence for a genetic component in adult-onset myopia within a large cohort study of white twins. He and colleagues 39 estimated a high genetic contribution to axial length, anterior chamber depth, and angle opening distance in twins from the Guangzhou Twin Registry.
Myopia Loci
See e-Supplement 1 (available at jaapos.org) for several recently identified loci with linkage to myopia (Pang CP, Lam CY, Tam PO, et al. Poster 1397–2007, American Society of Human Genetics, 2007). 40 – 49
Candidate Gene Studies
The list of hypothesized candidate genes for myopia is based largely on the current understanding of the pathophysiology of syndromic myopia. 50 The majority of the work examining the relationship between myopia and individual polymorphisms in candidate genes has been performed on single candidates at a time, to the exclusion of other independent or interacting genes. The results for many of the candidate myopia genes are promising and may have biological plausibility, but most are not conclusive and could not be replicated in other studies. Functional SNP effects have not been implicated for all of these candidate genes, and it is unclear how ethnic differences play a role in the degree of associative significance. 50
Animal Models
One impediment to correlating genotypic data with tissue histopathology in human myopia is that the tissue of interest (ie, retina/ sclera) cannot be directly sampled. Animal models of myopia have been developed to be used as surrogates, although it is unclear how correlative induced myopia in animals may be to physiologic myopia in humans. Animal studies over the past 30 years in juvenile and newborn monkey, tree shrew, and chick models have revealed an active emmetropization mechanism that normally achieves and maintains a match of the ocular AL to the eye’s optical power so that the photoreceptors are in focus for distant objects. Thus genes expressed in retina, RPE, choroid, and/or sclera that control this emmetropization process, if irregularly expressed, could cause the eye to elongate and become myopic. The emerging picture is one of complex interaction, in which mutations in several genes likely act in concert. The majority of myopia cases are not caused by defects in structural proteins, but by defects involving the control of structural proteins.
The first knock-out mouse model for relative myopia was based on form-deprivation experiments in chickens, mice, and rhesus macaque monkeys. 51 This model involved the immediate early gene transcription factor ZENK (also known as Egr-1), which is up-regulated in retinal amacrine cells when axial eye growth is inhibited by positive lens wear, and is down-regulated when axial growth is enhanced by negative lenses, suggesting that ZENK is linked to an axial eye growth inhibitory signal. ZENK knockout mice had longer eyes and a myopic shift relative to heterozygous and wild-type mice with identical genetic background. 51
Zhou and colleagues 52 provided a helpful record of refraction, corneal curvature, axial components, and the correlations between refraction and ocular growth during emmetropization in C57BL/6 mice. Refraction was most myopic at day 25 then shifted in the hyperopic direction to reach a peak at 47 days.
Hyperopic defocus, where the conjugate point of the object of regard is behind the retina, was been shown in early animal models to stimulate eye growth that moves the retina toward the conjugate point. Myopic defocus was reported to inhibit axial elongation, more robustly in the chick eye than in the mammalian eye, with the choroid of the chick pushing the retina forward toward the myopic focal point. 53 , 5 , Later studies showed that animal eyes respond bidirectionally to a level of defocus greater than its distance from emmetropia. The bidirectional modulation of eye growth by hyperopic and myopic defocus in disparate species suggests that the same may occur in children. 55 – 57
Although three earlier studies in humans suggest that increases in accommodative lag occur before the onset of myopia, 58 , 59 the Collaborative Longitudinal Evaluation of Ethnicity and Refractive Error (CLEERE) Study concluded that increased hyperopic defocus from accommodative lag may be a consequence rather than a cause of myopia. 60 Increased accommodative lag relative to model estimates of lag in emmetropes did not occur in children who became myopic before the onset of myopia or during the year of onset. 60
Evidence that excessive accommodation does not cause myopia includes the fact that visual deprivation myopia can be induced in the young of many animal species, including primates after ciliary ganglion destruction, Edinger-Westphal nucleus destruction or following optic nerve section. 61 , 62 , Recovery from refractive errors induced by the wearing of minus or plus lenses can also occur accommodation has been surgically abolished. 61 , 62
More recently, studies on infant monkeys suggest that the peripheral retina can play an important role in modulating overall eye growth and axial refraction. 63 – 65 While studies 66 , 67 strongly suggest an association between relative peripheral hyperopia and the development of myopia in humans, a causative role for the former has yet to be confirmed. It may still be that the relative peripheral hyperopia observed in eyes that become myopic is simply associated with the more prolate or less oblate shape of the eye.
Interventions to Retard the Progression of Myopia
Many interventions aimed at slowing myopia progression have been proposed; however, few have been subjected to the scientific rigors of randomized controlled trials. The rest of the studies have been retrospective case series, nonrandomized controlled trials and uncontrolled clinical trials.
Atropine Eyedrops
Atropine is a nonselective muscarinic antagonist. It was first used for myopia treatment by Wells 1 in nineteenth century. Subsequent studies have shown some clinical effect on the progression of myopia in children. 68 – 70 In addition, atropine inhibits myopia in tree shrew and monkey myopia models and blocks form deprivation myopia or lens induced myopia in chicks. 71 – 74
In contrast to the mammalian eye, the avian eye contains striated intraocular muscle and atropine has neither mydriatic nor cycloplegic effect in birds, indicating a non accommodative mechanism for anti-myopia activity of atropine in chick. 73 – 75
Unlike early atropine treatment studies for myopia which had various methodological shortcomings such as regular and detailed follow-up examinations, absence of appropriate clinical controls, absence of masking of participants and investigators, the Atropine in the Treatment of Myopia study (ATOM) was a randomized, double-masked, placebo-controlled trial involving 400 Singapore children 74 – 77 ( Figure 1 ). It showed that 1% atropine eyedrops instilled nightly in one eye over a 2-year period reduces myopic progression significantly in children by 77% (0.28 D in the control group versus 1.2 D in the atropine group). The atropine group’s mean axial length remained essentially unchanged, whereas the placebo group’s mean axial length increased 0.39 ± 0.48 mm. The topical atropine was well tolerated. Multifocal electroretinogram testing of the ATOM study subjects at 2 or 3 months after cessation of atropine or placebo treatment revealed no significant effect on retinal function. 78

Forest-plot of randomized clinical trials of interventions to retard the progression of myopia: weighted mean difference (95% CI). A, Atropine eyedrops versus control; B, Pirenzepine 2% gel versus control; C, Bifocals versus single-vision lenses; D, Progressive additional lenses versus single-vision lenses; E, Contact lenses versus single-vision lenses; F, RGP versus soft contact lenses.
Side effects of atropine include photophobia due to mydriasis and decreased near vision due to cycloplegia. 1 As a result, if atropine is used in both eyes, the patient needs photochromatic, progressive additional lenses. The ATOM study 78 reported no systemic side effects although possibilities include dry eye, dry mouth, dry throat, flushed skin, constipation and difficulty with micturition. In addition, there appears to be an initial increased rate of myopia progression following the cessation of atropine treatment in the ATOM study subjects (−1.14 ± 0.8 D in the atropine group vs −0.38 ± 0.39 D in the control group, p < 0.0001). 80 This “rebound” phenomenon is probably related to the strong cycloplegic effects of atropine. However, after 3 years of participation in the trial (with 2 years on atropine treatment), eyes randomized to atropine have less severe myopia than other eyes. Spherical equivalent was −4.29 ± 1.67 D in the atropine treated eyes compared with −5.22 ± 1.38 D in the placebo-treated eyes ( p < 0.0001).
Other issues to be addressed include determining the mechanism of action in retardation of myopia progression and possible long-term effects like ultraviolet light induced damage to lens and retina. The psychological effects of such a regimen on children need to be taken into consideration too. Finally, the optimal concentration and desired duration of drug application need to be established.
Currently, the decision to use atropine eye drops for retarding myopia progression should strike a balance between known short-term benefits of reducing myopia progression and the risks of side effects of atropine. It may be a viable option for children with rapidly progressive, high myopia and strong family history of high myopia and its comorbidities such as retinal detachment.
Pirenzepine 2% gel
This is a selective M-1 antagonist with a long history of oral use to treat dyspepsia and pediatric endocrine disorders in Europe and Asia. 81 Unlike atropine, which is equipotent in binding to M3 (accommodation and mydriasis) and M1 muscarinic receptors, pirenzepine is relatively selective for the M1 muscarinic receptor and thus is less likely than atropine to produce mydriasis and cycloplegia. 82 In the US pirenzepine 2% gel applied twice a day slowed myopia progression over 2 year (0.58 D vs 0.99 D). 83 In Asia the mean increases in myopia were 0.47 D, 0.70 D, 0.84 D in twice daily–once nightly control groups over 1 year. 84 Pirenzepine 2% gel applied twice a day and nightly reduced myopia progression by 50% and 44%, respectively ( Figure 1 ). Currently development of pirenzepine as an anti-myopia therapeutic has ceased due to regulatory and financial obstacles.
Optical Treatment
Reports in animal and human studies suggest that increased retinal defocus is a factor in the pathogenesis of myopia. 85 – 87 In humans high accommodative lag has been associated with myopia. 87 It was postulated that bifocals or multifocals could provide clear vision over a range of viewing distances, reduce retinal defocus and slow the progression of myopia. However, randomized, clinical trials in the US, Finland, and Denmark showed no significant slowing of myopia ( Figure 1 ). 88 – 91
Progressive Additional Lenses
The use of progressive addition lenses (PALs) has produced relatively small treatment effects ( Figure 1 ). 92 – 93 , 34 In particular, the correction of myopia evaluation trial (COMET, a multicenter, randomized, double-masked clinical trial, concluded that the overall adjusted 3-year treatment effect of 0.20 ± 0.08 D was statistically significant ( p = 0.004) but not clinically meaningful. 34 All the treatment effect occurred in the first year. Additional analyses showed that there were more significant treatment effects in children with larger lags of accommodation in combination with near esophoria (0.64 ± 0.21 D), shorter reading distances (0.44 ± 0.20 D), or lower baseline myopia (0.48 ± 0.15 D). 34 Though statistically significant, these differences over a 3-year period are not clinically meaningful.
Contact Lenses
Although anecdotal reports have suggested that the use of soft contact lenses speeds up myopia progression resulting in a phenomenon of “myopic creep,” randomized trials reported no significant difference in progression between soft contact lens and spectacle wearers. 94 – 96 On the other hand, soft contact lenses and rigid gas permeable lenses (RGP) were not shown to be effective in retarding myopia progression either ( Figure 1 ). 95 – 97
In the Contact Lens and Myopia Progression study, subjects were randomized to wear either RGP or soft contact lenses for 3 years. 98 Results showed a statistically significant difference in myopia progression in the RGP vs. soft lens group (−1.56 ± 0.95 D for RGP wearers vs −2.19 ± 0.89 D for the soft lens group, p < 0.001) with most of the treatment effect found in the first year ( Figure 1 ). 59 Corneal curvature steepened significantly less in the RGP group (0.62 ± 0.60 D) compared to the soft lens group (0.88 ± 0.57 D, p = 0.01). 98 Three-year axial elongation was not significantly different between treatment groups. These results suggest that the slowed myopia progression was mainly due to corneal flattening, which may be reversible with discontinuation of RGP lens wear. In the absence of differences in axial elongation, the authors concluded that RGP lenses was not effective for myopia control. 98
Orthokeratology
In overnight orthokeratology—also known as OOK, OK, ortho-k, and corneal reshaping—the patient wears reverse geometry lenses overnight to temporarily flatten the cornea and provide clear vision during the day without any glasses or contact lenses. 99 Reduction in the myopia (up to −6 D) is achieved by central corneal epithelial thinning, midperipheral epithelial, and stromal thickening. More than one hundred cases of severe microbial keratitis related to orthokeratology have been reported since 2001. 100
There is still no evidence for long-term efficacy of orthokeratology in reducing myopia progression. The often quoted Longitudinal Orthokeratology Research in Children involved 35 children in Hong Kong who wore OK lenses for 2 years. 101 Although results of the study showed that the axial length in the orthokeratology group increased by 0.29 mm versus 0.54 mm for the control group, a major scientific flaw was that control group was actually a historical control group of children wearing single vision lenses. More recently, the Corneal Reshaping and Yearly Observation of Nearsightedness (CRAYON) Pilot Study compared 28 subjects with corneal reshaping contact lenses with soft contact lens wearer from another myopia control trial. 63 The annual rate of change in axial length was 0.16 mm per year less ( p = 0.00004) for corneal reshaping lens wearer than soft contact lenses. However, limitations of this study included high drop out rate (30%), the choice of soft contact lenses as control group and the small numbers. 102 A gold standard randomized controlled trial with sufficient subject numbers still needs to be conducted to definitively determine whether orthokeratology is effective for slowing myopia progression
Undercorrection
The literature on myopigenesis suggests an active emmetropization mechanism regulated by optical defocus. Strong evidence is provided by compensatory ocular growth in response to lens-induced defocus in different species of animals. 103 A myopic defocus, where the optical image is formed in front of retina, results in a growth response toward hyperopia in animals. Only one masked, randomized clinical trial involving 94 children has been conducted to compare undercorrection by 0.75 D with full correction with single vision lenses. 104 Two-year progression in the fully corrected group was 0.77 D, significantly less than the 1.0 D in the undercorrected group ( p < 0.01) ( Figure 2 ). Contrary to animal studies, myopic defocus speeds up myopia progression rather than retarding it. This means that myopes may have an abnormal mechanism for detecting the direction of optical defocus of the retinal image.

Summary of forest plots of randomized clinical trials to retard myopia progression.
Part-time Lens Wear
Patterns of lens wear in myopes patients can vary from full-time wear, to the use of lenses for distance viewing only, to non-wear of prescribed lenses. Preliminary data of 43 subjects suggest that there is no effect of the pattern of lens wear on the progression of myopia. Three-year refractive shifts were not significantly different among the 4 groups: 105 (1) full-time wearers, (2) myopes who switched from distance to full-time wear, (3) distance wearers, and (4) nonwearers. A randomized clinical trial using a large sample of children randomly assigned to a lens wear regimen is warranted.
Commercial Products and Techniques
Neurovision.
The term perceptual learning describes a process whereby practicing certain visual tasks leads to an improvement in visual performance. Brain plasticity in visual functions has been shown in various studies. The NeuroVision technology is a noninvasive, patient specific, Internet-based perceptual learning program based on visual stimulation. 106 , 107 It facilitates neural connections at the cortical level using Gabor patches which are are local gray-level gratings with spatial frequencies of 1.5 to 12.0 cycles per degree (cpd) modulated from a background luminance of 40 cdm. They are widely used in visual neurosciences and have been shown to efficiently activate and match the shape of receptive fields in visual cortex. Gabor patches are used in different configurations, with different levels of spatial frequency, contrast, orientation, spatial location, distance, displacement, task order, exposure duration.
The patient is shown 2 consecutive displays in random order. Each display has some arrangement of Gabor patches with subtle differences. The patient is asked to identify the correct display as determined by the instructions for the specific task. If the patient answers correctly, the target contrast will be reduced and the task will become more difficult. On the other hand, incorrect answers will trigger the program to increase the contrast and the task becomes easier. NeuroVision improves neuronal efficiency and contrast sensitivity function by reducing the noise, increasing signal strength and thereby reducing the signal-to-noise ratio of neural activity in the primary visual cortex. 106 , 107
Although NeuroVision has been shown to improve unaided visual acuity and unaided contrast sensitivity in adults with low myopia, it does not alter refraction or accommodative amplitudes. 107 , 108 Children with highly progressive myopia often use undercorrected glasses and experience poor vision. A pilot study on 31 children aged 7 to 9 years showed that NeuroVision resulted in improvement in mean undercorrected visual acuities and mean undercorrected contrast sensitivity function (Chua WH, Hong CY, et al. Poster 52, 12th International Myopia Conference, 2009). After one year, the progression of myopia in this group was 0.5 D, which was less than the average progression in the age-matched normals from the Singapore Cohort Study of Risk Factors for Myopia (SCORM) study (0.944 D). A randomized controlled trial is needed to scientifically support these findings. In the meantime, NeuroVision should not be used for retardation or prevention of myopia.
“EyeRelax”
This is a microscope-like device purported to improve the vision of emmetropes, myopes, and even presbyopes, to prevent the worsening of myopia and to treat amblyopia. The retail price is around US$580. Users are to peer into the eye-pieces of the device for 5 minutes per eye, where they will see a kaleidoscope of brightly colored lights that focus and defocus. However, there is no is no evidence that it can retard the progression of myopia.
“Vision Therapy Eyewear”/Pinhole Glasses
These are black opaque lenses which have multiple small holes in them. A 10% to 20% improvement in vision—even elimination of myopia—is advertised. However, there is no evidence that this can retard myopia progression. What it actually uses is the pinhole effect where only coherent rays of light pass through. As the pinhole blocks most of the light rays, there is a smaller circle of blur on the retina. 108
Bates Method
Dr. William H Bates (1860–1931) attributed nearly all sight problems to habitual strain of the eyes and published a book entitled. The Bates method is based on his book The Cure of Imperfect Sight by Treatment without Glasses 109 and teaches techniques such as palming and sunning. The child is to register together with a parent for the workshop run by various companies, for a fixed sum of money. “Good habits of natural perfect sight” are taught, with some of the advertised benefits being “relaxed vision with better eye-mind coordination; improved memory and concentration; improved color vision and depth perception.” However, the principles behind the Bates technique are very different from conventional teaching and understanding. Bates’s anecdotal reports of improved vision have not been evaluated in trials.
Recommendations
The search for an effective intervention to slow the progression of myopia remains hampered by the lack of clear understanding of the exact pathogenesis of myopia. In searching for a clinically validated and effective therapy to retard myopia progression, future research should take into consideration the role of environmental factors to genetic influences, such as interactions of early-age near-work or outdoor activity and genotype. Consideration also needs to be given to the identification of phenotypes indicating etiologically homogeneous subgroups, for example, early age-of-onset, with/without retinal degenerative changes, or classification by individual response to treatments that reduce accommodation to near objects, such as progressive addition lens use.
Steady progress has been made in the field of human myopia genetics, but there is much still to be done. For example, no results have implicated more that just a single gene, or have expanded into an analysis of a specific pathway. This may implicate additional genes in a pathway also shown to be risk factors for myopia in validation studies, and point to potential haplotype-specific treatments. No candidate genes have been shown to account for even a modest fraction of the familial risk of myopia, and most of the data are conflicting about whether a true association exists. Candidate gene studies underscore that myopia is very complex, in fact, so complex that single candidate gene studies are unlikely to demonstrate the type of relationships needed to account for the majority of susceptibility genes. Thus there is a need for a genome-wide approach, incorporating candidate genes but not restricted to the study of candidate genes, to explore the relative contributions and interactions between known candidate genes and possibly novel genes in increased myopia susceptibility.
Randomized clinical trials of a variety of interventions such as bifocal lenses, progressive additional lenses and contact lenses have yielded disappointing results of results of marginal clinical significance ( Figure 2 ). To date, topical atropine 1% is the most promising. However, its short-term side effects, such as photophobia, have decreased compliance and possible long-term effects like ultraviolet light induced damage to lens and retina have limited its clinical use for retarding myopia progression. It is a viable option for high risk children with rapidly progressive myopia. Although topical pirenzepine, a selective M1-muscarinic antagonist, has also been shown to retard myopia progression, it is no longer commercially available. With regard to optical correction, current evidence suggests full correction. As for commercial devices, the anecdotal accounts of “improvement” or reduction of myopia may be related to pseudomyopia which often occurs in children because of their high accommodative facility.
As several large studies conducted in different parts of the world have reported that the prevalence of myopia in children with more outdoor activity hours is lower than in children with fewer hours, a promising but yet to be tested therapy could just simply be increased outdoor play. Peripheral refraction interventions to retard myopia progression may also be possible in the near future.
Supplementary Material
Acknowledgments.
Funded by Research to Prevent Blindness, Inc, the National Eye Institute, National Institutes of Health, and the Singapore Ministry of Health private grant.
Publisher's Disclaimer: This is a PDF file of an unedited manuscript that has been accepted for publication. As a service to our customers we are providing this early version of the manuscript. The manuscript will undergo copyediting, typesetting, and review of the resulting proof before it is published in its final citable form. Please note that during the production process errors may be discovered which could affect the content, and all legal disclaimers that apply to the journal pertain.
Presented as a workshop at the 35th Annual Meeting of the American Association for Pediatric Ophthalmology and Strabismus, San Francisco, California, April 17 to 21, 2009.
The authors have no conflicts of interest to report.
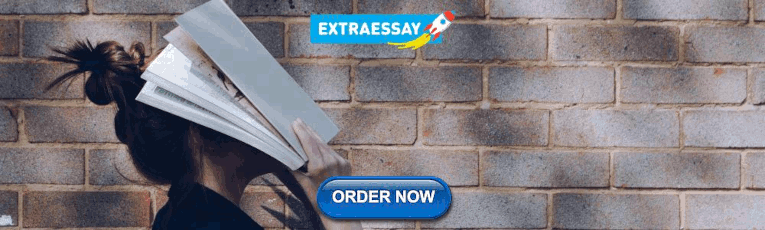
IMAGES
VIDEO
COMMENTS
Shih et al., in a randomized 18-month clinical research on a total of 227 school children aged 6-13 years, studied myopia progression reduction by using atropine and/or multifocal lenses. They discovered that combining 0.5% atropine with multifocal lenses slows the development of myopia as compared to using multifocal lenses alone or a ...
This paper presents a current overview on the concepts of myopia, and is expected to summarize updates on myopia control methods. Keywords: atropine, myopia, myopia control ... Researches and clinicians needs to remain updated with recent advancements in myopia and its control methods. Huge research gaps exist and hundreds of questions remains ...
1.2.2. Myopia is produced in the laboratory. Harvard and Massachusetts Institute of Technology research. A crucial paper coauthored by Nobel Prize winner Torsten Wiesel reported that by chance in their experiments suturing the eyelids closed produced myopia in monkeys, although they did not know why. 156 Feedback theory predicted that myopia could be produced experimentally, so a connection ...
Myopia, also known as short-sightedness or near-sightedness, is a very common condition that typically starts in childhood. Severe forms of myopia (pathologic myopia) are associated with a risk of other associated ophthalmic problems. ... 2 Myopia Research Group, Singapore Eye Research Institute, Singapore, Singapore. 3 Duke-NUS Medical School ...
A total of 22 509 papers related to myopia were published from the WOS Core Collection database. These papers were sorted by TCs, and the top 100 papers in the field of myopia were identified. ... and identified the 100 most influential articles that have had the greatest impact on myopia research in the past few decades. This study emphasizes ...
For example, the data in Table 7 can be used to calculate the benefit of 2 D of control in a patient destined to have myopia of -7 D (8.35 - 6.19 = 2.16 years of visual impairment) or a benefit of 3 D of slowing in a patient who would otherwise have -6 D of myopia (7.22 - 4.42 = 2.8 years of visual impairment).
Myopia is a leading cause of visual impairment and has raised significant international concern in recent decades with rapidly increasing prevalence and incidence worldwide. Accurate prediction of ...
Myopia, also known as short-sightedness or near-sightedness, is a very common condition that typically starts in childhood. ... UK Biobank and the Genetic Epidemiology Research on Adult Health and ...
The definitions of myopia and high myopia vary across the selected prevalence studies. Of the 145 articles included in this study, the most common definition of myopia was spherical equivalent of −0.50 diopter (D) or less (58.7%), with 29.0% using less than −0.50 D, 5.0% using −1.00 D or less or less than −1.00 D (all studies of adults), 2.9% using −0.75 D or less or less than −0. ...
Abstract. Myopia, a pandemic refractive error, is affecting more and more people. The progression of myopia could cause numerously serious complications, even leading to blindness. This review summarizes the epidemiological studies on myopia after 2018 and analyzes the risk factors associated with myopia. The prevalence of myopia varies in ...
In this systematic review and meta-analysis we searched MEDLINE and Embase, and manually searched reference lists for primary research articles investigating smart device (ie, smartphones and tablets) exposure and myopia in children and young adults (aged 3 months to 33 years) from database inception to June 2 (MEDLINE) and June 3 (Embase), 2020.
Background: Myopia is a global public health problem affecting quality of life and work productivity. Data is scarce regarding the effects of near work on myopia. Providing a larger meta-analysis with life-long perspective, including adults and occupational exposure seemed needed. Methods: We searched PubMed, Cochrane Library, Embase and Science Direct for studies reporting myopia prevalence ...
Abstract. Myopia is a dynamic and rapidly moving field, with ongoing research providing a better understanding of the etiology leading to novel myopia control strategies. In 2019, the International Myopia Institute (IMI) assembled and published a series of white papers across relevant topics and updated the evidence with a digest in 2021.
This third series of white papers published in 2023 highlights other key areas of myopia research and management, namely, evidence around onset, progression, and management of myopia in the less typical young adult 16 and pediatric (infant and pre-school children less than 5 years of age) 17 populations, the role of the choroid in eye growth ...
advancing myopia research. A series of 'white papers' on topics critical to progressing the capacity of the eye care sector to address the rapidly increasing prevalence of myopia, have now been developed by over 85 international experts through the IMI.
Importance Reducing myopia progression can reduce the risk of associated ocular pathologies.. Objective To evaluate whether spectacle lenses with higher lenslet asphericity have a higher myopia control efficacy throughout 2 years.. Design, Setting, and Participants This double-masked randomized clinical trial was conducted between July 2018 and October 2020 at the Eye Hospital of Wenzhou ...
Myopia is an increasingly serious health issue among children and adolescents worldwide. This study investigated the situation related to myopia among students in Chengdu, a city in western China, and analyzed the prevalence of myopia spectacle wear and myopia full-correction and their influencing factors to understand the current status of myopia prevention.
Starting with a World Health Organization (WHO) global scientific meeting on myopia at BHVI, Sydney in 2015, the International Myopia Institute was then formed to continue the efforts of the meeting and address the challenges of myopia and high myopia. Full IMI White Papers and translated IMI Clinical Summaries are available below.
This third series of white papers published in 2023 highlights other key areas of myopia research and management, namely, evidence around onset, progression, and management of myopia in the less typical young adult 16 and pediatric (infant and pre-school children less than 5 years of age) 17 populations, the role of the choroid in eye growth control and myopia management, 18 and a detailed ...
Purpose: Myopia or short-sightedness: first manifests in school-children (early-onset), and also in young adults (late-onset). Pathological myopia can lead to stretching of the eyeball to the point of blindness from a detached retina. This title presents a preliminary understanding of endocrine, juxtacrine, and paracrine factors: hoping to stimulate further research and enable early ...
INTRODUCTION. Myopia, or nearsightedness, is the most common human eye disorder in the world, and is a significant global public health concern. (1-2) Along with cataract, macular degeneration, infectious disease, and vitamin A deficiency, myopia is one of the most important causes of visual impairment worldwide.(2-3) Severe or high-grade myopia is a leading cause of blindness because of ...
(1) Background: Myopia is one of the leading causes of visual impairment. Visual work and usage of electronic devices are known risk factors of myopia. Many education systems were forced to apply online and hybrid teaching methods, to reduce the number of new cases of COVID-19. Medical students are a population well-known for intense visual work in the form of learning; (2) Methods: Visual ...
Increased time outdoors. Earlier research found that increased participation in weekly sports and outdoor activities was associated with a lower risk of developing myopia.5 Later, the protective role of outdoor time against myopia came to prominence in the Sydney Myopia Study.6 Epidemiological studies spanning diverse geographical locations have subsequently supported this conclusion.7 ...
Purpose: To assess the efficacy and safety of repeated low-level red-light (RLRL) therapy in myopia control in children. Design: Multicenter, randomized, parallel-group, single-blind clinical trial. Participants: Two hundred sixty-four eligible children 8 to 13 years of age with myopia of cycloplegic spherical equivalent refraction (SER) of -1.00 to -5.00 diopters (D), astigmatism of 2.50 D or ...
The top 10 cited papers mainly focused on the epidemiology of myopia. Previous research emphasized myopia-associated experimental animal models, while recent keywords include "SMILE" and "myopia control" with the stronger burst, indicating a shift of concern from etiology to therapy and coincided with the global increment of incidence.
This third series of white papers published in 2023 highlights key areas of myopia research and management which have been gaining interest. These include: Emerging evidence for roles of the choroid in both myopia development and myopia control. The growing evidence in this field warrants further attention, particularly for clinicians who may ...
Rsearchers have developed the first 3D printable ocular resins, marking a significant breakthrough in manufacturing specialist lenses for implantation in the human eye.
Myopia, or near-sightedness, is the state of refraction in which parallel rays of light are brought to focus in front of the retina of a resting eye. 1 It is measured by the spherical power in diopters of the diverging lens needed to focus light onto the retina, which can be expressed as the spherical equivalent (SE), that is, sphere + half negative cylinder.