Thank you for visiting nature.com. You are using a browser version with limited support for CSS. To obtain the best experience, we recommend you use a more up to date browser (or turn off compatibility mode in Internet Explorer). In the meantime, to ensure continued support, we are displaying the site without styles and JavaScript.
- View all journals
- Explore content
- About the journal
- Publish with us
- Sign up for alerts
- Published: 02 November 2020
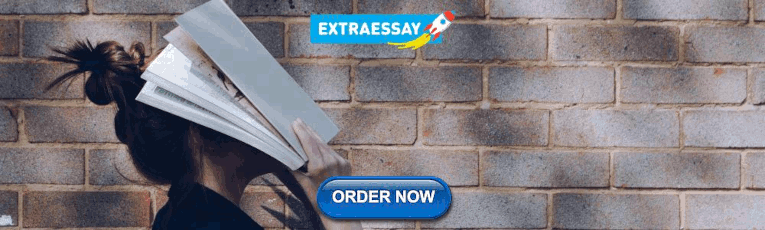
Translational research is all-encompassing and lets everyone be a researcher
- Eleanor J. Molloy 1 , 2 , 3 , 4 , 5 &
- Cynthia F. Bearer 6 , 7
Pediatric Research volume 90 , pages 2–3 ( 2021 ) Cite this article
3271 Accesses
5 Citations
3 Altmetric
Metrics details
Translational research
Biomedical research can be categorized based on its aim: (1) to determine the underlying nature of things (basic research) and (2) to understand the underlying nature of things in order to be able to improve human health (translational research). There are a diverse number of definitions of translational research, which usually includes an all-encompassing overview of all biomedical research from basic translational science to clinical implementation. 1 The National Center for Advancing Translational Sciences (NCATS’) definition of translation is broad and inclusive: “translation is the process of turning observations in the laboratory, clinic and community into interventions that improve the health of individuals and the public—from diagnostics and therapeutics to medical procedures and behavioural changes.” 1 This definition technically applies to much of the research in biomedical sciences ranging from preliminary laboratory-based research to epidemiology and implementation science. The core message is that research with the aim of improving health can ultimately be translated from the bench to patient care. 2 We therefore realized in Pediatric Research that defining a subset of articles as a category termed translational research was incorrect and removed this term. Instead, all articles published in Pediatric Research represent translational research and categorized based on methodology: basic translational research, clinical translational research, or population translational research, with translational research as the overriding theme of the journal (Fig. 1 ).
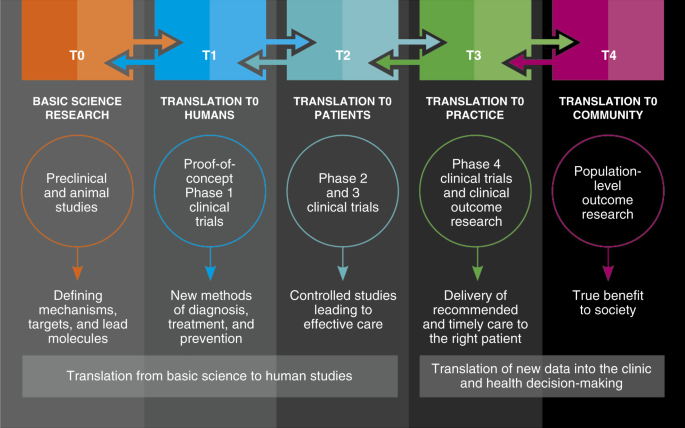
Adapted from image by Macmillan Publishers Ltd. Nature Medicine (Blumberg et al., copyright 2012).
Everyone is a researcher
There is a huge value for all healthcare personnel and families to participate in research. Although career development is another important motivation, the advantages are the ability to cope with rejection and there is increasing evidence that being research-active avoids burnout and builds resilience. 3 , 4 It is postulated that research develops a deep sense of purpose of oneself and allows one the chance to change and improve outcomes. Everyone can be involved in research, either in their daily clinical practice including quality improvement or to laboratory-based or epidemiologic research. Translational research from basic to epidemiologic has been the basis of the seven great advances in pediatrics, including: immunizations to prevent disease, reducing sudden infant death, cure for acute lymphoblastic leukemia, surfactant therapy for premature infants, preventing vertical HIV transmission, improving life expectancy for children with sickle cell anemia and cystic fibrosis, and saving lives with car seats and seat belts. 5
Cheng et al. 6 have also suggested that the future of pediatric research advancements, including new immunizations, cancer immunotherapy, genomics discoveries, identification of early antecedents of adult health, impact of specific social–environmental influences on biology and health, quality improvement science, and implementation and dissemination research to reduce global poverty, would be based on translational research. Further research and programmatic investment early in the life course is essential.
Children and family involvement in research
There are many motivations for families to participate in research. Healthcare workers are predominantly altruistic to get evidence-based answers for clinical applications that can improve outcomes for children and families. 7 Patients’ and public involvement (PPI) is a way of involving anyone not professionally interested or experienced in health care into research. The engagement of patients and the public into research is termed participatory research.
Upholding the rights of the child enshrined in the United Nation’s Convention on the Rights of the Child (UN CRC), which states that all children have the right to an opinion and to express their views. They all should have the right to be informed and give their opinion about the world around them. 8
Developing PPI using resources, such as medical conferences, publishing, editorial, and research boards, are vital. The James Lind alliance is an organization with the idea of bringing together clinicians, patients, and carers to discuss research priorities and to develop the top 10 research priorities ( http://www.jla.nihr.ac.uk/ ). Initiatives for children to lead research projects have been developed. This research projects also increase awareness about the benefits of research and clinical trials. Child-led research has several prominent examples including RCTS by children ( https://www.hrb-tmrn.ie/public-engagement/start-competition/ ) to develop better quality evidence, and more applicable and impactful research. In these PPI, children are involved in all aspects of the project from its inception to publication and implementation and are a source of empowerment.
Developing research capacity
In Pediatric Research , we have started a series of editorials on career development at all stages from early career investigator to senior roles. Training for research is vital. National and international resources are available. For example, in the UK the academic toolkit ( https://academictoolkit.org/ ) has been developed in conjunction with the Royal College of Pediatrics and Child Health (RCPCH) for pediatricians in training. There are multiple resources for families and patients who want to be involved in PPI, including training courses ( http://www.icphr.org/ppiphr-courses.html ) and the RCPCH patient research charter. 9 Improvement in research infrastructure and training were noted by the RCPCH in their document “Turning the Tide 5 years on,” but they noted more development was needed (Turing the Tide 5 years on… https://www.rcpch.ac.uk/sites/default/files/2018-03/turning_the_tide_-_five_years_on_2018-03.pdf ).
Database development, artificial intelligence, and big data will be major resources when combined with new discovery and human capacity in research. The concept that all healthcare professionals are researchers helps to ensure the integration of research in health care. Healthcare professionals have a privileged position to improve family outcomes through translational research and the engagement of all stakeholders.
Austin, C. P. Translation translation. Nat. Rev. Drug Discov. 17 , 455–456 (2018).
Article CAS Google Scholar
Rubio, D. M. et al. Defining translational research: implications for training. Acad. Med. 85 , 470–475 (2010).
Article Google Scholar
Halliday, L., Walker, A., Vig, S., Hines, J. & Brecknell, J. Grit and burnout in UK doctors: a cross-sectional study across specialties and stages of training. Postgrad. Med. J. 93 , 389–394 (2017).
Galaiya, R., Kinross, J. & Arulampalam, T. Factors associated with burnout syndrome in surgeons: a systematic review. Ann. R. Coll. Surg. Engl. 102 , 401–407 (2020).
Cheng, T. L., Bogue, C. W. & Dover, G. J. The next 7 great achievements in Pediatric Research. Pediatrics 139 , e20163803 (2017).
Cheng, T. L. et al. Seven great achievements in pediatric research in the past 40 y. Pediatr. Res. 80 , 330–337 (2016). pmid:27556199.
Molloy, E. J., Mader, S., Modi, N. & Gale, C. Parent, child and public involvement in child health research: core value not just an optional extra. Pediatr. Res. 85 , 2–3 (2019).
Molloy, E. J. Dr Janusz Korczak: paediatrician, children’s advocate and hero. Pediatr. Res. 86 , 783–784 (2019).
Hunter, L. et al. Advancing child health research in the UK: the Royal College of Paediatrics and Child Health Infants’ Children’s and Young People’s Research Charter. Arch. Dis. Child. 102 , 299–300 (2017).
Download references
Acknowledgements
This work was supported by National Children’s Research Centre, Crumlin, Dublin, Ireland and Health Research Board Ireland (to E.J.M.) and NIH/NICHD P01HD085928 (to C.F.B.).
Author information
Authors and affiliations.
Discipline of Paediatrics, Trinity College, The University of Dublin, Dublin, Ireland
- Eleanor J. Molloy
Children’s Health Hospital (CHI) at Tallaght, Tallaght University Hospital, Dublin, Ireland
Trinity Translational Medicine Institute (TTMI), St. James Hospital and Trinity Research in Childhood Centre (TriCC), Dublin, Ireland
Department of Neonatology, CHI at Crumlin, Dublin, Ireland
Department of Paediatrics, Coombe Women’s and Infant’s University Hospital, Dublin, Ireland
Department of Pediatrics, Division of Neonatology, Rainbow Babies and Children’s Hospital, Cleveland, OH, USA
Cynthia F. Bearer
Case Western Reserve University School of Medicine, Cleveland, OH, USA
You can also search for this author in PubMed Google Scholar
Contributions
E.J.M. and C.F.B.: substantial contributions to conception and design, revising the article critically for important intellectual content; final approval of the version to be published.
Corresponding author
Correspondence to Eleanor J. Molloy .
Ethics declarations
Competing interests.
The authors declare no competing interests.
Additional information
Publisher’s note Springer Nature remains neutral with regard to jurisdictional claims in published maps and institutional affiliations.
Rights and permissions
Reprints and permissions
About this article
Cite this article.
Molloy, E.J., Bearer, C.F. Translational research is all-encompassing and lets everyone be a researcher. Pediatr Res 90 , 2–3 (2021). https://doi.org/10.1038/s41390-020-01225-4
Download citation
Received : 15 September 2020
Accepted : 15 September 2020
Published : 02 November 2020
Issue Date : July 2021
DOI : https://doi.org/10.1038/s41390-020-01225-4
Share this article
Anyone you share the following link with will be able to read this content:
Sorry, a shareable link is not currently available for this article.
Provided by the Springer Nature SharedIt content-sharing initiative
This article is cited by
Incorporating anti-racist principles throughout the research lifecycle: a position statement from the society of general internal medicine (sgim).
- Christopher J. Gonzalez
- Sudarshan Krishnamurthy
- Himali Weerahandi
Journal of General Internal Medicine (2024)
Multisystem inflammatory syndrome in children (MIS-C) and neonates (MIS-N) associated with COVID-19: optimizing definition and management
- Natasha Nakra
- Satyan Lakshminrusimha
Pediatric Research (2023)
Doing a PhD: ten golden rules
- E. J. Molloy
- C. F. Bearer
Pediatric Research and COVID-19: the changed landscape
- C. B. Bearer
Pediatric Research (2022)
Quick links
- Explore articles by subject
- Guide to authors
- Editorial policies

- Your Stories
Evidence Based Living
Bridging the gap between research and real life
- In The Media
- The Learning Center
- Cornell Cooperative Extension
- Youth Development
- Health and Wellness
What is translational research?

Cornell’s College of Human Ecology is pursuing a translational research model to better link social and behavioral science research to extension and outreach, creating a more seamless link between science and service. But the question arises: What is “translational research?”
Evidence-Based Living sat down with Wethington to talk about the growing field of translational research.
To start off, what exactly is translational research?
Many definitions have been given for translational research, but the definition I like best is that it is a systematic effort to convert basic research knowledge into practical applications to enhance human health and well being.
Translational research was designed for the medical world. It emerged in response to concern over the long time lag between scientific discoveries and changes in treatments, practices, and health policies that incorporate the new discoveries.
What is applied research, and how does it differ?
Translational research is broader than the traditional term “applied research.” Applied research is any research that may possibly be useful for enhancing health or well-being. It does not necessarily have to have any effort connected with it to take the research to a practical level.
For example, an applied research study might analyze longitudinal data that tracks participants’ health and social relationships. The researchers would report their findings in an academic journal.
But in translational research, the same study would include some “action steps.” The researchers would partner with a community and ask for ideas about how their findings might apply there. Together, they would come up with an intervention plan that would also include scientific evaluation of its effectiveness.
Why are social science researchers slower to adopt these models compared to the medical community?
I think the answer to this question is that researchers have followed where the money has been allocated. The opportunities for social and behavioral scientists have not been established as rapidly.
More recently, three major government institutions have been funding projects that emphasize public health outreach using translational research – the Centers for Disease Control, the National Institutes of Health and the National Institute on Aging. All three have been establishing translational research centers across the country, primarily focused on underserved communities and health disparities.
Thus, social scientists are only now being encouraged to take part. More recently economic stimulus funds dispersed the National Institute of Health funded a number of translational research projects headed by social scientists, including three funded at Cornell. I predict that soon there will be social scientists engaged in translational research across the country, not just at funded centers.
What are the benefits of moving toward translational research?
For researchers, there is benefit to being affiliated with a center that provides seed funding for projects, methodological assistance, advice on developing proposals and experience in getting community input into research projects.
For universities, translational research centers provide a tactical advantage for attracting more funding. Translational research centers also provide a way for universities to meet public service goals in their strategic plans.
For communities, translational research provides opportunities to make a difference in their own communities. As part of one of the Cornell centers, we engaged public service agency directors in events where they could contribute to our research agenda. With a stake in the research, communities feel that they are making a valued and important contribution. We heard over and over from the community members that this was a real source of pride and accomplishment for them.
How can extension programs participate?
One way local extension programs can participate in translational research is to take part in community stakeholder groups that meet with researchers who are designing intervention and prevention research programs. Typically, a wide variety of stakeholders need to be engaged. County Cooperative Extension offices have many collaborative relationships in their counties and can work with researchers to make contacts.
Typically, local extension professionals do not have time to engage in research themselves. Yet they have valuable experience that can be shared. This makes Cooperative Extension an ideal contributor for implementing programs.
Nice reading! Thank you
A remarkable extraordinary read, ideally other huge schools can take an interest in something like this.
Quite the great read, hopefully other large schools are able to participate in something like this.
Speak Your Mind Cancel reply
A project at…, evidence-based blogs.
- Bandolier Evidence-Based Health Care
- British Psychological Society Research Digest
- Economics in Cooperative Extension
- EPB Exchange
- New Voices for Research
- Research Blogging
- Trust the Evidence
Subscribe by e-mail
Please, insert a valid email.
Thank you, your email will be added to the mailing list once you click on the link in the confirmation email.
Spam protection has stopped this request. Please contact site owner for help.
This form is protected by reCAPTCHA and the Google Privacy Policy and Terms of Service apply.
Return to top of page
Copyright © 2024 · Magazine Theme on Genesis Framework · WordPress · Log in
- Letter to the Editor
- Open access
- Published: 27 May 2024
Analyzing global research trends and focal points of pyoderma gangrenosum from 1930 to 2023: visualization and bibliometric analysis
- Sa’ed H. Zyoud ORCID: orcid.org/0000-0002-7369-2058 1 , 2
Journal of Translational Medicine volume 22 , Article number: 508 ( 2024 ) Cite this article
55 Accesses
Metrics details
To the Editor, I read with great interest the publication entitled “An approach to the diagnosis and management of patients with pyoderma gangrenosum from an international perspective: results from an expert forum” [ 1 ]. Pyoderma gangrenosum is an ulcerative, cutaneous condition with distinctive clinical characteristics first described in 1930 [ 2 ]. Due to the importance of the subject, this published study was searched in databases, and I did not find any bibliometric studies on this topic. In recent years, researchers have successfully applied bibliometric analysis in various domains, contributing to the development of novel theories and assessing research frontiers, including in the dermatology field. Nonetheless, comprehensive bibliometric analyses of P. gangrenosum have not been performed. This study addresses this gap by conducting a thorough bibliometric analysis in the field of P. gangrenosum at the global level. The goal is to assist researchers in swiftly grasping the knowledge structure and current focal points in the field, generating new research topic ideas, and enhancing the overall quality of research on P. gangrenosum.
This bibliometric analysis sought to delineate research endeavors concerning P. gangrenosum, pinpoint the primary contributing countries, and discern prevalent topics within this domain. Using a descriptive cross-sectional bibliometric methodology, this study extracted pertinent documents from the Scopus database covering the period from 1930 to December 31, 2023. The search strategy included keywords related to ‘pyoderma gangrenosum.’ VOSviewer software (version 1.6.20) was used to illustrate the most recurring terms or themes [ 3 ]. The scope of the retrieved documents was restricted to including only journal research articles while ignoring other forms of documents.
Overall, 4,326 papers about P. gangrenosum were published between 1930 and 2023. Among these were 3,095 (71.54%) original papers, 548 (12.67%) letters, 477 (11.03%) reviews, and 206 (4.76%) other kinds of articles, such as conference abstracts, editorials, or notes. With 3,454 publications, English was the most often used language, followed by French ( n = 253), German ( n = 190), and Spanish ( n = 163), accounting for 93.85% of all related publications.
Figure 1 shows the distribution of these publications. Between 1930 and 2023, there were steadily more publications on P. gangrenosum (R 2 = 0.9257; P value < 0.001). Growth trends and productivity trends in P. gangrenosum-related publications have been influenced by developments in medical research, clinical practice and patient care [ 4 , 5 ]. All of these factors have advanced our knowledge of the condition, enhanced our methods of treatment, and helped to create standardized findings for clinical studies.
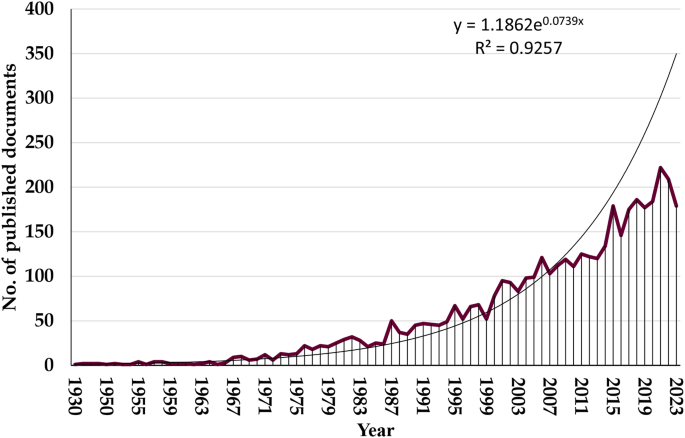
Annual growth of published research related to P. gangrenosum (1930–2023)
The top 10 countries with the most publications on P. gangrenosum are listed in Table 1 . These are the USA ( n = 1073; 24.80%), the UK ( n = 345; 7.98%), Japan ( n = 335, 7.74%), and Germany ( n = 296; 6.84%). With 65 articles, the Mayo Clinic in the USA led the institutions; Oregon Health & Science University in the USA and Università degli Studi di Milano in Italy followed with 60 articles each.
To create a term co-occurrence map in VOSviewer 1.6.20, terms had to appear in the title and abstract at least forty times by binary counting. The network was visualized by building the map using terms with the highest relevance scores. Large bubbles for often cooccurring terms and close spacing between terms with high similarity were guaranteed by the algorithm. The larger circles in Fig. 2 A represent frequently occurring terms in titles and abstracts. Four primary topic clusters—“Treatment modalities” (green cluster), “epidemiology and clinical presentation” (blue cluster), “improved diagnostic methods” (red cluster), and “the links between P. gangrenosum and other morbidities such as inflammatory bowel disease or autoimmune conditions” (yellow cluster)—are distinguished by color.
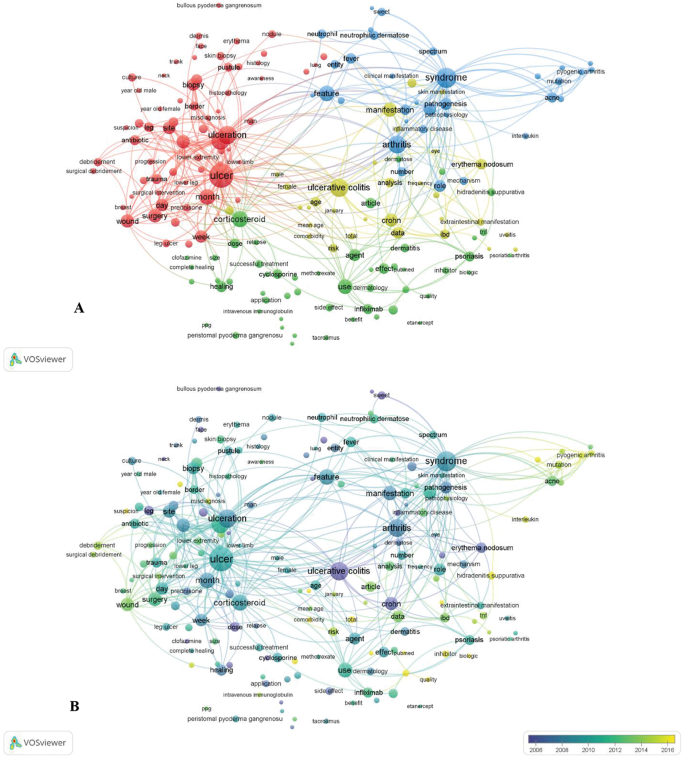
Mapping of terms used in research on P. gangrenosum. A : The co-occurrence network of terms extracted from the title or abstract of at least 40 articles. The colors represent groups of terms that are relatively strongly linked to each other. The size of a term signifies the number of publications related to P. gangrenosum in which the term appeared, and the distance between two terms represents an estimated indication of the relatedness of these terms. B : Mapping of terms used in research on P. gangrenosum. The terms “early” (blue) or “late” (red) years indicate when the term appeared
Interestingly, after 2012, terms related to “treatment modalities” and “epidemiology and clinical presentation” have gained more attention than in the past, which focused on “improved diagnostic methods” and “the links between P. gangrenosum and other morbidities such as inflammatory bowel disease or autoimmune conditions” (pre-2012). Figure 2 B shows this tendency.
In conclusion, there has recently been an increase in P. gangrenosum research, especially in the last decade. The current focus of research is on treatment challenges, obstacles to diagnosis, and connections to underlying diseases. Furthermore, efforts are being made to create core outcome sets and standardized diagnostic criteria for clinical trials. These patterns demonstrate continuous attempts to comprehend, identify, and treat this illness with greater effectiveness. This recent increase in research has important implications for clinical practice. Clinicians can improve patient care by remaining current in emerging trends and areas of interest. Moreover, an in-depth analysis of previous studies can identify knowledge gaps, directing future research efforts toward the most important issues. In the end, a deeper comprehension of the body of research can result in better clinical judgment based on best practices, which could enhance patient outcomes and advance the dermatological field.
Data availability
This published article contains all the information produced or examined in this research. Additional datasets utilized during this study can be obtained from the corresponding author.
Haddadin OM, Ortega-Loayza AG, Marzano AV, Davis MDP, Dini V, Dissemond J, Hampton PJ, Navarini AA, Shavit E, Tada Y, et al. An approach to diagnosis and management of patients with pyoderma gangrenosum from an international perspective: results from an expert forum. Arch Dermatol Res. 2024;316(3):89.
Article PubMed Google Scholar
Brunsting LA. Pyoderma (Echthyma) Gangrenosum. Arch Derm Syphilol. 1930;22(4):655–80.
Article Google Scholar
van Eck NJ, Waltman L. Software survey: VOSviewer, a computer program for bibliometric mapping. Scientometrics. 2010;84(2):523–38.
Kridin K, Cohen AD, Amber KT. Underlying systemic diseases in Pyoderma Gangrenosum: a systematic review and Meta-analysis. Am J Clin Dermatol. 2018;19(4):479–87.
McKenzie F, Arthur M, Ortega-Loayza AG. Pyoderma Gangrenosum: what do we know now? Curr Dermatol Rep. 2018;7(3):147–57.
Download references
Acknowledgements
The author thanks An-Najah National University for all the administrative assistance during the implementation of the project.
No support was received for conducting this study.
Author information
Authors and affiliations.
Department of Clinical and Community Pharmacy, College of Medicine and Health Sciences, An-Najah National University, Nablus, 44839, Palestine
Sa’ed H. Zyoud
Clinical Research Centre, An-Najah National University Hospital, Nablus, 44839, Palestine
You can also search for this author in PubMed Google Scholar
Contributions
Sa’ed H. Zyoud significantly contributed to the conceptualization and design of the research project, overseeing data management and analysis, generating figures, and making substantial contributions to the literature search and interpretation. Furthermore, Sa’ed H. Zyoud authored the manuscript, which he reviewed and approved as the sole author.
Corresponding author
Correspondence to Sa’ed H. Zyoud .
Ethics declarations
Ethics approval and consent to participate.
The ethics committee’s approval was unnecessary because the study did not include human interactions.
Consent for publication
Not applicable.
Competing interests
The authors declare that they have no competing interests.
Additional information
Publisher’s note.
Springer Nature remains neutral with regard to jurisdictional claims in published maps and institutional affiliations.
Rights and permissions
Open Access This article is licensed under a Creative Commons Attribution 4.0 International License, which permits use, sharing, adaptation, distribution and reproduction in any medium or format, as long as you give appropriate credit to the original author(s) and the source, provide a link to the Creative Commons licence, and indicate if changes were made. The images or other third party material in this article are included in the article’s Creative Commons licence, unless indicated otherwise in a credit line to the material. If material is not included in the article’s Creative Commons licence and your intended use is not permitted by statutory regulation or exceeds the permitted use, you will need to obtain permission directly from the copyright holder. To view a copy of this licence, visit http://creativecommons.org/licenses/by/4.0/ . The Creative Commons Public Domain Dedication waiver ( http://creativecommons.org/publicdomain/zero/1.0/ ) applies to the data made available in this article, unless otherwise stated in a credit line to the data.
Reprints and permissions
About this article
Cite this article.
Zyoud, S.H. Analyzing global research trends and focal points of pyoderma gangrenosum from 1930 to 2023: visualization and bibliometric analysis. J Transl Med 22 , 508 (2024). https://doi.org/10.1186/s12967-024-05306-4
Download citation
Received : 10 May 2024
Accepted : 14 May 2024
Published : 27 May 2024
DOI : https://doi.org/10.1186/s12967-024-05306-4
Share this article
Anyone you share the following link with will be able to read this content:
Sorry, a shareable link is not currently available for this article.
Provided by the Springer Nature SharedIt content-sharing initiative
Journal of Translational Medicine
ISSN: 1479-5876
- Submission enquiries: Access here and click Contact Us
- General enquiries: [email protected]

- SPORE Program Announcement
- SPORE Pre-Application Materials
- SPORE Grant Application Forms
- Research Resources
- SPORE Co-Funding and Partnerships
- Distribution of Grants
- SPORE Advances
- Translational Research Advances
- SPORE Investigator Meetings
Welcome to the Translational Research Program (TRP)
The Translational Research Program (TRP) is committed to reducing cancer incidence and mortality and improving survival and quality of life for cancer patients. TRP uses advances in basic sciences to develop new approaches for the prevention, diagnosis, and treatment of cancer by fostering interdisciplinary investigations and coordinating the resources of NCI with those of academia, industry, and nonprofit organizations and foundations.
These objectives are accomplished by:
- Supporting the Specialized Programs of Research Excellence (SPOREs) to translate novel scientific discoveries into clinical testing, including early-phase clinical trials
- Encouraging a multidirectional approach to translational research
- Promoting research in high incidence and rare cancers in adults and children
- Facilitating the cross-fertilization of ideas, leveraging resources, and ensuring access of resources to projects and investigators to bring discoveries from the laboratory to the clinic in the most efficient manner
- Supporting additional grant mechanisms for translational research
TRP is the home of the SPOREs — the Specialized Programs of Research Excellence - a cornerstone of NCI's efforts to promote collaborative, interdisciplinary translational cancer research. SPORE grants involve both basic and clinical/applied scientists working together and support projects that will result in new and diverse approaches to the prevention, early detection, diagnosis, and treatment of human cancers.
Each SPORE is focused on a specific organ site, a group of highly related cancers, or a common pathway or theme that ties together the cancers under study. SPOREs are designed to enable the rapid and efficient movement of basic scientific findings into clinical settings, as well as to determine the biological basis for observations made in individuals with cancer or in populations at risk for cancer. SPOREs are required to reach a human endpoint within the 5-year funding period. Currently, 21 organ sites, systems, and pathway-specific themes are represented in the SPORE portfolio, including: brain, breast, cancer health disparities, cervical, endometrial, epigenetics, gastrointestinal, head and neck, hyperactive RAS, liver, kidney, leukemia, lung, lymphoma, myeloma, , ovarian, pancreatic, prostate, radiation sensitivity, sarcoma, and skin.
The objective for all SPOREs is to reduce cancer incidence and mortality and to improve survival and quality of life for cancer patients. SPOREs encourage the advice of patient advocates in SPORE activities. Prospective applicants are encouraged to contact TRP Program Officials for advice prior to submission. The current Program Announcement for submitting applications can be found here .
TRP currently uses the P50, U54, and P20 funding mechanisms for the SPORE program. In addition, TRP staff currently oversee a relatively smaller number of R21, R01, and R50 translational research grants. The breakdown of 2023 grants across organ sites and thematic SPOREs can be found here.
TRP also manages grants that are part of special initiatives when those grants are translational and are connected to the themes of currently funded SPOREs. An example is the R50 Research Specialist Career Awards where the applicant is integral to the work of a funded SPORE.
TRP is also involved in the following DCTD Activities:
- Small Cell Lung Cancer Consortium (SCLC-C)
- Pancreatic Stromal Reprogramming Consortium (PSRC)
- The Integrated Canine Data Commons
- Longitudinal Studies in Dogs with Cancer as a Comparative Oncology Model
Future Directions
Following are descriptions of TRP initiatives planned for future years:
- Encourage research that will enable precision medicine approaches, such as genomic and proteomic-based diagnostic technologies, that will allow cancer patients with specific molecular alterations to receive the most effective treatments
- Increase translational cancer research areas that are underrepresented in the NCI portfolio: pancreatic cancer, endometrial cancer, pediatric cancers, and cancers that display health disparities
- Advance studies on the dynamic relationship between tumors and cells/mediators in the microenvironment to make a difference in the diagnosis and treatment of cancer, particularly in the area of immunotherapy
- Advance the goals of translational research by facilitating collaborations between SPOREs and other NCI-funded mechanisms such that discoveries can move quickly and seamlessly along pathways from the laboratory to Phase 1 and Phase 2 trials and beyond, with strong correlative study and biomarker support.
NCI Center to Reduce Cancer Health Disparities (CRCHD)
In partnership with NCI Center to Reduce Cancer Health Disparities (CRCHD) , the TRP supports feasibility and planning activities (P20) to develop translational research programs focused on cancer health disparities research with the expectation that the research programs will become competitive for a full Specialized Programs of Research Excellence (SPORE) awards.
TRP Priorities
Current priorities include translational research in:
- Cancer health disparities
- Endometrial cancer
- Pancreatic cancer
- Pediatric cancers
SPOREs and P20s by State
Spores by organ location.
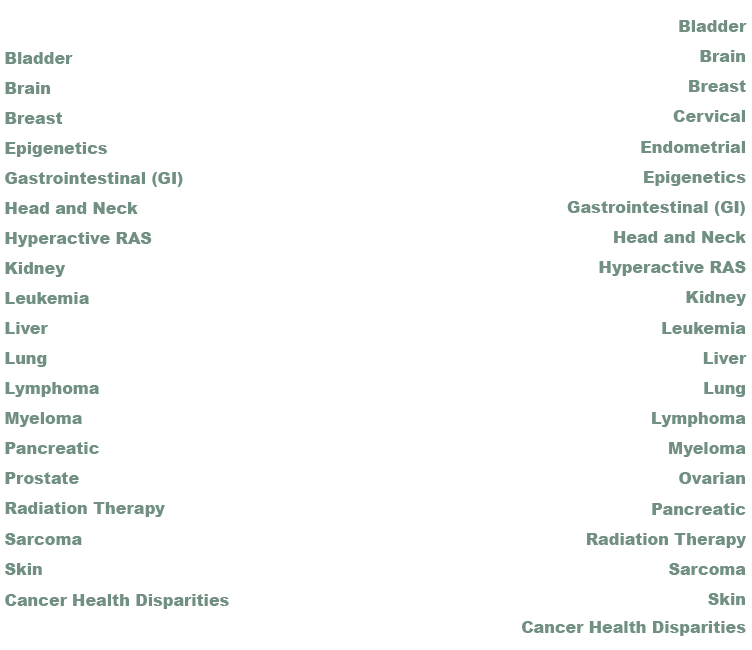
Quick Links
- Contact TRP
- Accessibility
- Disclaimer Policy
- HHS Vulnerability Disclosure
- U.S. Department of Health and Human Services
- National Institutes of Health
- National Cancer Institute
- Alzheimer's disease & dementia
- Arthritis & Rheumatism
- Attention deficit disorders
- Autism spectrum disorders
- Biomedical technology
- Diseases, Conditions, Syndromes
- Endocrinology & Metabolism
- Gastroenterology
- Gerontology & Geriatrics
- Health informatics
- Inflammatory disorders
- Medical economics
- Medical research
- Medications
- Neuroscience
- Obstetrics & gynaecology
- Oncology & Cancer
- Ophthalmology
- Overweight & Obesity
- Parkinson's & Movement disorders
- Psychology & Psychiatry
- Radiology & Imaging
- Sleep disorders
- Sports medicine & Kinesiology
- Vaccination
- Breast cancer
- Cardiovascular disease
- Chronic obstructive pulmonary disease
- Colon cancer
- Coronary artery disease
- Heart attack
- Heart disease
- High blood pressure
- Kidney disease
- Lung cancer
- Multiple sclerosis
- Myocardial infarction
- Ovarian cancer
- Post traumatic stress disorder
- Rheumatoid arthritis
- Schizophrenia
- Skin cancer
- Type 2 diabetes
- Full List »
share this!
May 29, 2024
This article has been reviewed according to Science X's editorial process and policies . Editors have highlighted the following attributes while ensuring the content's credibility:
fact-checked
peer-reviewed publication
trusted source
Proof-of-concept study pioneers new brain imaging technique through a transparent skull implant
by Keck School of Medicine of USC
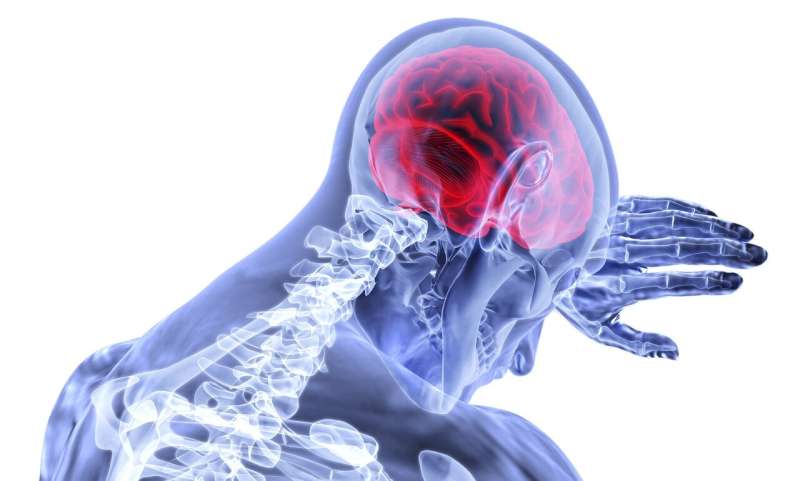
In the first study of its kind, researchers from the Keck School of Medicine of USC and the California Institute of Technology (Caltech) designed and implanted a transparent window in the skull of a patient, then used functional ultrasound imaging (fUSI) to collect high-resolution brain imaging data through the window.
Their preliminary findings , published in Science Translational Medicine , suggest that this sensitive, non-invasive approach could open new avenues for patient monitoring and clinical research , as well as broader studies of how the brain functions.
"This is the first time anyone had applied functional ultrasound imaging through a skull replacement in an awake, behaving human performing a task," said Charles Liu, MD, Ph.D., a professor of clinical neurological surgery, urology and surgery at the Keck School of Medicine and director of the USC Neurorestoration Center.
"The ability to extract this type of information noninvasively through a window is pretty significant, particularly since many of the patients who require skull repair have or will develop neurological disabilities. In addition, 'windows' can be surgically implanted in patients with intact skulls if functional information can help with diagnosis and treatment."
The research participant, 39-year-old Jared Hager, sustained a traumatic brain injury (TBI) from a skateboarding accident in 2019. During emergency surgery , half of Hager's skull was removed to relieve pressure on his brain, leaving part of his brain covered only with skin and connective tissue. Because of the pandemic, he had to wait more than two years to have his skull restored with a prosthesis.
During that time, Hager volunteered for earlier research conducted by Liu, Jonathan Russin, MD, associate surgical director of the USC Neurorestoration Center, and another Caltech team on a new type of brain imaging called fPACT.
The experimental technique had been done on soft tissue, but could only be tested on the brain in patients like Hager who were missing a part of their skull. When the time came for implanting the prosthesis, Hager again volunteered to team up with Liu and his colleagues, who designed a custom skull implant to study the utility of fUSI—which cannot be done through the skull or a traditional implant—while repairing Hager's injury.
Before the reconstructive surgery, the research team tested and optimized fUSI parameters for brain imaging, using both a phantom (a scientific device designed to test medical imaging equipment) and animal models. They then collected fUSI data from Hager while he completed several tasks, both before his surgery and after the clear implant was installed, finding that the window offered an effective way to measure brain activity.
Functional brain imaging, which collects data on brain activity by measuring changes in blood flow or electrical impulses, can offer key insights about how the brain works, both in healthy people and those with neurological conditions.
But current methods, such as functional magnetic resonance imaging (fMRI) and intracranial electroencephalography (EEG) leave many questions unanswered. Challenges include low resolution, a lack of portability or the need for invasive brain surgery. fUSI may eventually offer a sensitive and precise alternative.
"If we can extract functional information through a patient's skull implant, that could allow us to provide treatment more safely and proactively," including to TBI patients who suffer from epilepsy, dementia, or psychiatric problems, Liu said.
A new frontier for brain imaging
As a foundation for the present study, Liu has collaborated for years with Mikhail Shapiro, Ph.D. and Richard Andersen, Ph.D., of Caltech, to develop specialized ultrasound sequences that can measure brain function, as well as to optimize brain-computer interface technology, which transcribes signals from the brain to operate an external device.
With these pieces in place, Liu and his colleagues tested several transparent skull implants on rats, finding that a thin window made from polymethyl methacrylate (PMMA)—which resembles plexiglass—yielded the clearest imaging results. They then collaborated with a neurotechnology company, Longeviti Neuro Solutions, to build a custom implant for Hager.
Before surgery, the researchers collected fUSI data while Hager did two activities: solving a "connect-the-dots" puzzle on a computer monitor and playing melodies on his guitar. After the implant was installed, they collected data on the same tasks, then compared the results to determine whether fUSI could provide accurate and useful imaging data.
"The fidelity of course decreased, but importantly, our research showed that it's still high enough to be useful," Liu said. "And unlike other brain-computer interface platforms, which require electrodes to be implanted in the brain, this has far less barriers to adoption."
fUSI may offer finer resolution than fMRI and unlike intracranial EEG, it does not require electrodes to be implanted inside the brain. It is also less expensive than those methods and could provide some clinical advantages for patients over non-transparent skull implants, said Russin, who is also an associate professor of neurological surgery at the Keck School of Medicine and director of cerebrovascular surgery at Keck Hospital of USC.
"One of the big problems when we do these surgeries is that a blood clot can form underneath the implant, but having a clear window gives us an easy way to monitor that," he said.
Refining functional ultrasound technology
In addition to better monitoring of patients, the new technique could offer population-level insights about TBI and other neurological conditions. It could also allow scientists to collect data on the healthy brain and learn more about how it controls cognitive, sensory, motor and autonomic functions.
"What our findings show is that we can extract useful functional information with this method," Liu said. "The next step is: What specific functional information do we want, and what can we use it for?"
Until the new technologies undergo clinical trials, fUSI and the clear implant are experimental. In the meantime, the research team is working to improve their fUSI protocols to further enhance image resolution . Future research should also build on this early proof-of-concept study by testing more participants to better establish the link between fUSI data and specific brain functions, the researchers said.
"Jared is an amazing guy," said Liu, who is continuing to collaborate with the study participant on refining new technologies, including laser spectroscopy, which measures blood flow in the brain. "His contributions have really helped us explore new frontiers that we hope can ultimately help many other patients."
Explore further
Feedback to editors

Tobacco firms invest billions in medical products: Call for journals to ban research by the firms, their subsidiaries
11 hours ago

New avenues to developing personalized treatments for schizophrenia
12 hours ago

Study: Access to targeted lung cancer drug is cost-prohibitive globally

Researchers identify factors that heighten risk for catheter-associated urinary tract infections and sepsis

'Sticky and strain-gradient artificial epineurium' can heal severed nerves in only one minute, study claims
13 hours ago

Could ultra-processed foods be associated with your insomnia?

Tiny worm helps uncover long-lasting prenatal effects from amphetamines

PTSD, anxiety is rising among college students

Study confirms effectiveness of 'watch-and-wait' approach to prostate cancer
14 hours ago

Cause of common type of heart failure may be different for women and men
Related stories.

Doctors can now watch spinal cord activity during surgery
Mar 7, 2024

New approach to brain imaging leaves skulls intact in mice
Sep 5, 2022

Musk's Neuralink says issue in brain implant fixed
May 9, 2024

Musk says patient moves cursor with brain implant
Feb 21, 2024

Ultrasound enables less-invasive brain-machine interfaces
Nov 30, 2023

Neuralink shows quadriplegic playing chess with brain implant
Mar 21, 2024
Recommended for you

How does the word 'not' affect what we understand? Scientists find negation mitigates our interpretation of phrases
16 hours ago

In the brain at rest, study indicates neurons rehearse future experience

Study suggests astrocytes induce sex-specific effects on memory
18 hours ago

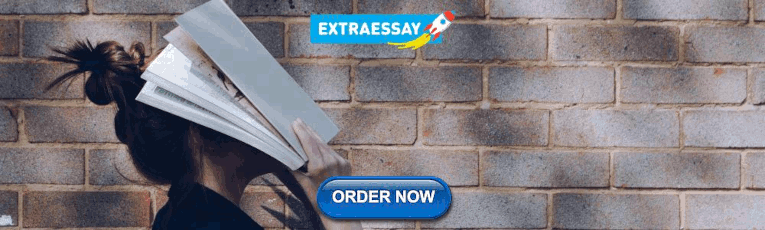
A multimodal approach to better predict recovery in patients with disorders of consciousness
21 hours ago

Too much or too little: The impact of protein dosage on fetal development
19 hours ago
Let us know if there is a problem with our content
Use this form if you have come across a typo, inaccuracy or would like to send an edit request for the content on this page. For general inquiries, please use our contact form . For general feedback, use the public comments section below (please adhere to guidelines ).
Please select the most appropriate category to facilitate processing of your request
Thank you for taking time to provide your feedback to the editors.
Your feedback is important to us. However, we do not guarantee individual replies due to the high volume of messages.
E-mail the story
Your email address is used only to let the recipient know who sent the email. Neither your address nor the recipient's address will be used for any other purpose. The information you enter will appear in your e-mail message and is not retained by Medical Xpress in any form.
Newsletter sign up
Get weekly and/or daily updates delivered to your inbox. You can unsubscribe at any time and we'll never share your details to third parties.
More information Privacy policy
Donate and enjoy an ad-free experience
We keep our content available to everyone. Consider supporting Science X's mission by getting a premium account.
E-mail newsletter

Official websites use .gov
A .gov website belongs to an official government organization in the United States.
Secure .gov websites use HTTPS
A lock ( ) or https:// means you've safely connected to the .gov website. Share sensitive information only on official, secure websites.

Translation Research
Translation research explores how scientific work moves into practice and benefits society. Although this new field of research has not received much attention in occupational safety and health, it can have a valuable impact on workers everywhere. Today’s workplaces need research findings and products that can improve the quality of life, health, and work. Through translation research, NIOSH studies the process, drivers, and barriers for turning knowledge into practical applications that create these improvements for the safety and health of workers.
Translation researchers apply scientific investigative approaches to study how the outputs of basic and applied research can be effectively translated into practice and have an impact. This includes studying how knowledge and interventions are spread, accepted, applied, and institutionalized.
Activities in translation research range across the basic-to-applied continuum, and studies referred to as intervention research and translation research often overlap. NIOSH delineates the two in this way: intervention research involves improving an intervention or designing a new one, whereas translation research involves studying processes for putting research outputs into practice or use. These processes can include activities such as efficacy research (testing interventions in a realistic setting) and effectiveness research (testing interventions in a wide range of settings). Translation research also includes limited proof-of-concept testing and large-scale studies of research outputs and their impact.
Translation research has four stages (Table 1):
- Stage 1, development translation research , studies how a discovery made in a laboratory, field or pilot study or findings of risks, can move into a potential health and safety application to be tested.
- Stage 2, testing translation research , assesses the value of a new finding, invention, process, training program, or intervention. It looks for larger-scale workplace safety and health practices aimed at a specific work sector or across two or more work sectors.
- Stage 3, institutionalization translation research , studies how evidence-based technology and recommendations become well-accepted workplace safety and health practices that are communicated and used on a large scale.
- Stage 4, evaluation translation research , explores the “real world” health benefits and effects of moving these discoveries and interventions into large-scale practice. This phase examines impact over time through ongoing surveillance and evaluation.
Table 1. Examples of Translation Research for Different Types of Hazards
* Stage 0 is the basic or applied research finding that becomes the focus for study in Stages 1–4 of the translation research framework. It is not part of that framework.
The translation research process should be a never-ending loop of research and translation, allowing for ongoing integration of effective approaches. This focus on improving the implementation of science shows NIOSH’s value as the nation’s primary occupational safety and health research institute. Translation research findings add great value to occupational safety and health research by helping us understand how science can better achieve practical benefits and what factors stand in the way of scientific work leading to useful outcomes. Translation research reveals how discoveries and guidance improve the lives of workers.
The phrases translation research , translational research , and translational science have been used to describe the systematic effort to convert basic research outputs into practical applications to enhance human health and well-being. Generally, these uses of the phrase translational research refer to harnessing knowledge from basic medical science and bringing it into clinical practice (so-called bench-to-bedside models). In contrast, NIOSH defines the phrase translation research to refer to the study of the process of moving “research-to-practice-to-impact” and the methods, barriers and facilitators, context, and issues encountered in this process. However, in the scientific literature, the two descriptors of research—translation versus translational—overlap.
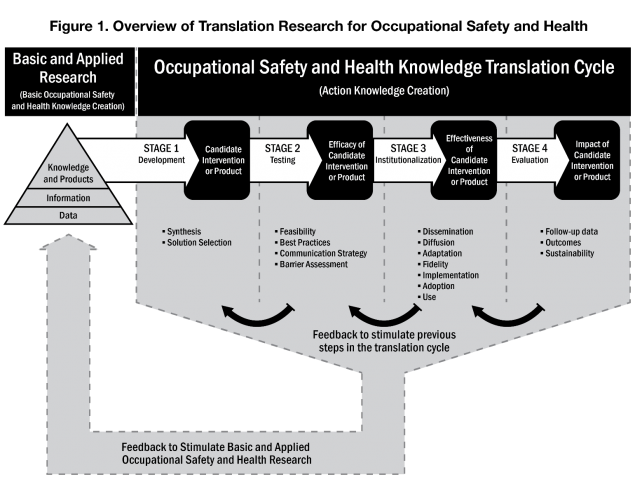
Source: Schulte et al. [2017]
In 2016 NIOSH developed a framework for a wide-ranging plan for translation research. The plan will help NIOSH study how to move research findings into practice as quickly as possible, to improve worker safety.
NIOSH created its Translation Research Program to nurture, promote, and coordinate translation research and to more effectively transform science into useful actions and products. Translation research explores the processes by which research findings are successfully implemented in the workplace.
Compared with other workplace safety and health efforts, translation research is a new field without a large base of scientific papers. The program builds on previous NIOSH health communication work and research into effective interventions. It also includes research on social and behavioral science topics and on how scientific innovations spread and become practical benefits to society.
The research-to-practice process starts with research and ends with useful innovations. The Translation Research Program’s efforts focus on the research-to-practice continuum to learn how to make any given step better and how to improve its impact. The goal is to develop relevant, widely applied knowledge that creates a safer and healthier work environment.
This work faces challenges, however:
- Identifying translation research priorities, to focus limited resources yet achieve maximum impact
- Focusing on how decision-makers get and use workplace safety and health information, as well as barriers that prevent such information from reaching those who need it
- Finding which approaches work best in making safety and health research products that will have the most effective benefits.
NIOSH continues to gather translation research, promote goals for internal research competitions, and develop the skills of staff. Its work outside the institute may include offering dedicated funding opportunities and developing and promoting training for translation research.
Publications which have guided the development of the NIOSH Translation Research Program include the following:
- Schulte PA; Cunningham TR; Nickels L; Felknor S; Guerin R; Blosser F; Chang C-C; Check P; Eggerth D; Flynn M; Forrester C; Hard D; Hudson H; Lincoln J; McKernan LT; Pratap P; Stephenson CM; Van Bogaert D; Menger-Ogle L [2017]. Translation research in occupational safety and health: a proposed framework . Am J Ind Med 2017 Dec; 60(12):1011-1022.
- Dugan, A.G. & Punnett, L. Dissemination and Implementation Research for Occupational Safety and Health. Occup Health Sci (2017) 1: 29.
- Tinc PJ, Gadomski A, Sorensen JA, Weinehall L, Jenkins P, Lindvall K. Adapting the T0-T4 implementation science model to occupational health and safety in agriculture, forestry, and fishing: A scoping review. Am J Ind Med. 2018;61:51–62.
- Khoury MJ, Gwinn M, Ioannidis JPA [2010]. The emergence of translational epidemiology: from scientific discovery to population health impact. American Journal Epidemiology 172: 517-524.
- Lucas DL, Kincl LD, Bovbjerg VE and Lincoln JM [2014]. Application of a translational research model to assess the progress of occupational safety research in the international commercial fishing industry. Safety Science 64:71-81.
- Ogilvie D, Craig P, Griffin S, Macintyre S and Wareham NJ [2009]. A translational framework for public health research. BMC Public Health 9(1):116.
- NIOSH Translation Research Roadmap and Implementation presentation to the Board of Scientific Counselors Meeting, March 2016
- Hager LD. BLS occupational hearing loss report for 2007. CAOHC Update. 2009; 21: 7-9.
- Berger EH, Franks JR, Behar A, et al. Development of a new standard laboratory protocol for estimating field attenuation of hearing protection devices. Part III. The validity of using subject-fit data. J Acoust Soc Am. 1998; 103: 665-672.
- Murphy WJ, Davis RR, Byrne DC, Franks JR. Advanced hearing protector study. Cincinnati, OH: U.S. Department of Health and Human Services, Centers for Disease Control and Prevention, National Institute for Occupational Safety and Health, NIOSH EPHB Report No. 312-11a; 2007. https://ntrl.ntis.gov/NTRL/dashboard/searchResults/titleDetail/PB2008113769.xhtml
- Stephenson CM, Stephenson MR. Hearing loss prevention for carpenters. Part 1-using health communication and health promotion models to develop training that works. Noise Health. 2011; 13: 113-121.
- Davies H, Marion S, Teschke K. The impact of hearing conservation programs on incidence of noise-induced hearing loss in Canadian workers. Am J Ind Med. 2008; 51: 923-931.
- Masterson EA, Deddens JA, Themann CL, Bertke S, Calvert GM. Trends in worker hearing loss by industry sector, 1981-2010. Am J Ind Med. 2015; 58: 392-401.
- Wagner GR. The inexcusable persistence of silicosis. Am J Public Health. 2005; 85: 1346-1347. https://www.ncbi.nlm.nih.gov/pmc/articles/PMC1615617/
- Cecala AB, O’Brien AD. Here comes the Helmet-CAM: A recent advance in technology can improve how mine operators investigate and assess respirable dust. Rock Prod. 2014; 117: 26-30.
- Haas EJ, Willmer D., Cecala AB. Formative research to reduce mine worker respirable silica dust exposure: A feasibility study to integrate technology into behavioral interventions. Pilot Feasibility Study. 2016; 2: 6.
- Reed WR, Kwitowski AJ, Helfrich WJ, Cecala AB, Joy GJ. Guidelines for performing a helmet-CAM respirable dust survey and conducting subsequent analysis with the Enhanced Video Analysis of Dust Exposures (EVADE) software. Pittsburgh, PA: U.S. Department of Health and Human Services, Centers for Disease Control and Prevention, National Institute for Occupational Safety and Health, DHHS, (NIOSH) Publication No. 2014-133; 2014. https://www.cdc.gov/niosh/mining/UserFiles/works/pdfs/2014-133.pdf
- Bourbonnais R, Comeau M, Vézina M. Job strain and evolution of mental health among nurses. J Occup Health Psychol. 1999; 4: 92-107.
- Bourbonnais R, Brisson C, Vinet A, Vézina M, Lower A. Development and implementation of a participative intervention to improve the psychosocial work environment and mental health in an acute care hospital. Occup Environ Med. 2006; 63: 326-334.
- Jimmieson NL, Hobman EV, Tucker MK, Bordia P. Change in psychosocial work factors predicts follow-up employee strain: An examination of Australian employees. J Occup Environ Med. 2016; 58: 1002-1013.
- Ruotsalainen JH, Verbeek JH, Mariné A, Serra C. Preventing occupational stress in healthcare workers. Cochrane Database Syst Rev. 2015; 4: D002892.
- Dollard M, Skinner N, Tuckey MR, Bailey T. National surveillance of psychosocial risk factors in the workplace: An international overview. Work Stress. 2997; 21: 1-29.
- NIOSH. Commercial fishing vessel shipper dies after being pulled into a deck winch-Alaska. Anchorage, AK: U.S. Department of Health and Human Services, Centers for Disease Control and Prevention, National Institute for Occupational Safety and Health, Fatality Assessment and Control Evaluation (FACE) Program, State FACE Reports-Alaska: Report No. FACE AK-95-23; 1995. https://www.cdc.gov/niosh/face/stateface/ak/95ak023.html
- Lincoln JM, Lucas DL, McKibbin RW, Woodward CC, Bevan JE. Reducing commercial fishing deck hazards with engineering solutions for winch design. J Safety Res. 2008; 39: 231-235.
- Lincoln JM, Woodward CC, King GW, Case SL, Lucas DL, Teske TD. Preventing fatal winch entanglements in the US southern shrimp fleet: A research to practice approach. J Safety Res. 2017; 60: 119-123.
- Levin JL, Gilmore K, Wickman A, et al. Workplace safety interventions for commercial fishermen of the gulf. J Agromedicine. 2016; 21: 178-189.
- Teske TD, Victoroff T. Increasing adoption of safety technologies in commercial fishing. Study in progress. Anchorage, AK: U.S. Department of Health and Human Services, Centers for Disease Control and Prevention, National Institute for Occupational Safety and Health (NIOSH); 2017.
- CDC. Fatal and nonfatal injuries involving fishing vessel winches- Southern shrimp fleet, United States, 2000-2011. MMWR. 2013; 62: 157-160. https://www.cdc.gov/mmwr/preview/mmwrhtml/mm6209a1.htm
Exit Notification / Disclaimer Policy
- The Centers for Disease Control and Prevention (CDC) cannot attest to the accuracy of a non-federal website.
- Linking to a non-federal website does not constitute an endorsement by CDC or any of its employees of the sponsors or the information and products presented on the website.
- You will be subject to the destination website's privacy policy when you follow the link.
- CDC is not responsible for Section 508 compliance (accessibility) on other federal or private website.

An official website of the United States government
The .gov means it’s official. Federal government websites often end in .gov or .mil. Before sharing sensitive information, make sure you’re on a federal government site.
The site is secure. The https:// ensures that you are connecting to the official website and that any information you provide is encrypted and transmitted securely.
- Publications
- Account settings
Preview improvements coming to the PMC website in October 2024. Learn More or Try it out now .
- Advanced Search
- Journal List
- HHS Author Manuscripts

Defining Translational Research: Implications for Training
Doris mcgartland rubio.
Associate Professor and Director of the Data Center, Center for Research on Health Care, Division of General Internal Medicine, Department of Medicine, School of Medicine, University of Pittsburgh, Pittsburgh, PA
Ellie E. Schoenbaum
Professor of Epidemiology & Population Health, Director Education and Career Development Core, Institute for Clinical and Translational Research, Institute for Clinical and Translational Research, Albert Einstein College of Medicine and Montefiore Medical Center, Bronx, NY
Linda S. Lee
Associate Director for the Clinical Research Training Program, Department of Biostatistics and Bioinformatics, School of Medicine, Duke University, Durham, NC
David E. Schteingart
Professor, Department of Internal Medicine, Division of Metabolism, Endocrinology and Diabetes; Co-Director, Education, Mentoring and Career Development Core, Michigan Institute for Clinical and Health Research; University of Michigan, Ann Arbor
Paul R. Marantz
Associate Dean for Clinical Research Education and Professor, Department of Epidemiology and Population Health, Albert Einstein College of Medicine and Montefiore Medical Center, Bronx, NY
Karl E. Anderson
Professor, Department of Preventive Medicine and Community Health, University of Texas Medical Branch, Galveston, TX
Lauren Dewey Platt
Executive Director, Scholars in Clinical Science Program, Harvard Catalyst/The Harvard Clinical and Translational Science Center, Harvard Medical School, Tosteson Medical Education Center, Boston, MA
Adriana Baez
Professor, Departments of Pharmacology and Otolaryngology-Head and Neck Surgery, University of Puerto Rico School of Medicine, San Juan, Puerto Rico
Karin Esposito
Assistant Professor of Clinical Psychiatry, Department of Psychiatry and Behavioral Sciences, Miller School of Medicine, University of Miami, Miami, FL
Because translational research is not clearly defined, developers of translational research programs are struggling to articulate specific program objectives, delineate the knowledge and skills (competencies) that trainees are expected to develop, create an appropriate curriculum, and track outcomes to assess whether program objectives and competency requirements are being met. Members of the Evaluation Committee of the Association for Clinical Research Training (ACRT) reviewed current definitions of translational research and proposed an operational definition to use in the educational framework. In this article, the authors posit that translational research fosters the multidirectional and multidisciplinary integration of basic research, patient-oriented research, and population-based research, with the long-term aim of improving the health of the public. The authors argue that the approach to designing and evaluating the success of translational training programs must therefore be flexible enough to accommodate the needs of individual institutions and individual trainees within the institutions but that it must also be rigorous enough to document that the program is meeting its short-, intermediate-, and long-term objectives and that its trainees are meeting preestablished competency requirements. A logic model is proposed for the evaluation of translational research programs.
The National Institutes of Health (NIH) has traditionally supported the training of basic and clinical scientists in a variety of disciplines. More recently, it has supported the training of scientists in translational research through the K30 and Clinical and Translational Science Award (CTSA) programs. 1 Because both basic research and clinical research are clearly defined, developers of programs to train individuals in these types of research have been able to articulate program objectives, delineate the knowledge and skills (i.e., competencies) that trainees are expected to develop, create an appropriate curriculum, and track outcomes to assess whether program objectives and competency requirements are being met. In contrast, because translational research is not clearly defined, developers of translational research programs are struggling with some of these processes.
As members of the Evaluation Committee of the Association for Clinical Research Training (ACRT), we began to address this problem by reviewing the definitions of different types of research. We then developed working definitions of translational research and associated terms. Here, we present our working definitions, discuss their implications for educational training programs, and offer a framework to guide institutions in developing processes of program evaluation.
Definitions of Basic Research and Basic Science
According to the American Cancer Society, basic science involves laboratory studies that provide the foundation for clinical research. 2 As one cancer center indicates, basic science entails gathering knowledge that is essential for applying discoveries to patient care. 3 However, in 1945, when the director of the US Office of Scientific Development and Research proposed the establishment of the National Science Foundation (NSF), he made the following distinction between basic research and applied research:
Basic research is performed without thought of practical ends. It results in general knowledge and an understanding of nature and its laws. This general knowledge provides the means of answering a large number of important practical problems, though it may not give a complete specific answer to any one of them. The function of applied research is to provide such complete answers. 4
The NSF definition thus identifies the main objective of basic research as the acquisition of knowledge without the obligation to apply it to practical ends.
Basic research training is pervasive in medical schools. With PhD programs offered in disciplines such as the biomedical sciences, computational biology, and neuroscience, the basic science field has been well established. Competencies within the basic sciences have been clearly defined, allowing for effective development and evaluation of educational programs. About 60% of the NIH budget is allocated for basic research, and most of the basic research funds go to PhD scientists. 5
Definitions of Clinical Research
In 1997, the NIH Director’s Panel on Clinical Research issued the following 3-part definition of clinical research:
- Patient-oriented research. Research conducted with human subjects (or on material of human origin such as tissues, specimens and cognitive phenomena) for which an investigator (or colleague) directly interacts with human subjects. Excluded from this definition are in vitro studies that utilize human tissues that cannot be linked to a living individual. Patient-oriented research includes: (a) mechanisms of human disease, (b) therapeutic interventions, (c) clinical trials, or (d) development of new technologies.
- Epidemiologic and behavioral studies.
- Outcomes research and health services research. 6
In 1998, the NIH introduced the Clinical Research Curriculum Award to “improve the quality of training in clinical research.” 7 Over 50 training programs were funded through this award, and many of these programs grant degrees in clinical research.
The NIH’s definition of clinical research has been widely accepted by institutions and programs and provides a common basis for the NIH-funded clinical research training programs. The definition has facilitated cross-program efforts to identify core competencies, best practices, and meaningful outcomes that are relevant across the broad spectrum of training in clinical research. This in turn has allowed program evaluators to develop useful assessment metrics to document the success of training programs.
Today, about 30% of the NIH budget is spent on clinical research. 5 However, some believe that this figure includes studies of animal models, in which case the actual support for clinical research would be much less. 5
Definitions of Translational Research
The definition of translational research is less clear than the definitions of basic and clinical research.
Although a Medline search indicates that the term translational research appeared as early as 1993, there were relatively few references to this term in the medical literature during the 1990s, and most references were to research about cancer. At the time, the literature on cancer tended to use the term translational research to refer to work spanning different types of research (e.g., immunology studies spanning basic and clinical research) or work spanning disciplines within a particular type of research (e.g., bench research involving molecular genetics and immunology). Today, the literature includes a plethora of attempts in various fields to define the term. 8
In a recent announcement about applying for a CTSA, the NIH offered the following definition:
Translational research includes two areas of translation. One is the process of applying discoveries generated during research in the laboratory, and in preclinical studies, to the development of trials and studies in humans. The second area of translation concerns research aimed at enhancing the adoption of best practices in the community. Cost-effectiveness of prevention and treatment strategies is also an important part of translational science. 9
According to this definition, translational research is part of a unidirectional continuum in which research findings are moved from the researcher’s bench to the patient’s bedside and community. In the continuum, the first stage of translational research (T1) transfers knowledge from basic research to clinical research, while the second stage (T2) transfers findings from clinical studies or clinical trials to practice settings and communities, where the findings improve health.
In a commentary published in 2008, Steven Woolf pointed out that “translational research means different things to different people” 10 (p211) and argued that the different types of translational research are too narrowly defined. In particular, he argued that if T2 research is going to result in the knowledge needed to improve health and the quality of life, then T1 research must include sciences related to populations (e.g., epidemiology, psychology, economics, and behavioral sciences). 10
When the Institute of Medicine (IOM) convened the Clinical Research Roundtable, the roundtable group developed a model for translational research that was highly aligned with the NIH definition. 11
Like the NIH and the IOM, the Translational Research Working Group of the National Cancer Institute (NCI) included both basic and clinical research in the T1 segment of the continuum: “Translational research transforms scientific discoveries arising from laboratory, clinical, or population studies into clinical applications to reduce cancer incidence, morbidity, and mortality.” 12 But given that basic research and clinical research involve inherently different knowledge sets and methodologies, including both of them in the same segment of the continuum (i.e., in T1) obscures the fact that multidisciplinary translational research can also occur at the interface of basic and clinical science.
A Framework to Design and Evaluate Translational Research Programs
As members of the ACRT Evaluation Committee, our goal was to develop a framework to assess translational research programs. We quickly realized, however, that disagreements over what is and is not included in the definition of translational research would make it difficult for us to define competency requirements and determine whether these requirements were being met. We therefore began discussing the definitions outlined above. We also consulted articles published on the topic of translational research 8 , 10 , 11 , 13 , 14 and data provided on the Web sites of the first 12 CTSA recipients. 15
Working definitions
We developed the following working definition of translational research:
Translational research fosters the multidirectional integration of basic research, patient-oriented research, and population-based research, with the long-term aim of improving the health of the public. T1 research expedites the movement between basic research and patient-oriented research that leads to new or improved scientific understanding or standards of care. T2 research facilitates the movement between patient-oriented research and population-based research that leads to better patient outcomes, the implementation of best practices, and improved health status in communities. T3 research promotes interaction between laboratory-based research and population-based research to stimulate a robust scientific understanding of human health and disease.
We believe that when T1 is conceptualized as the process of moving from bench to bedside, it represents a movement toward the goal of improved health. It may evoke the image of a patient receiving medical care or the image of a healthy individual benefiting from improvements in health care or public health. Alternatively, it may suggest that patient-oriented research (research at the bedside) is a key step toward improvement in the treatment or prevention of disease.
The model that we propose (see Figure 1 ) captures the dynamic interplay inherent in the concept of translational research. The model’s circular structure suggests that research is a continuing cycle, and its bidirectional arrows emphasize that new knowledge and hypotheses are generated at each step. Some basic research and population-based research is translational, but neither type of research is by definition translational. In contrast, patient-oriented research fundamentally addresses issues that have the potential to translate to clinical practice and therefore affect health. For these reasons, the model includes only part of basic research and population-based research within the circular structure but includes all of patient-oriented research within this structure.

Model for translational research, as proposed by the Evaluation Committee of the Association for Clinical Research Training.
The concept of basic research, as defined earlier, is generally well understood. The concepts of patient-oriented research and population-based research fall within the broader rubric of clinical research as defined by the NIH. We use the term patient-oriented research to refer to studies that include groups of patients or healthy individuals and are designed to understand the mechanisms of disease and health, to determine the effects of a treatment, or to provide a decision analysis of the care trajectories of patients. 16 Clinical trials are an example of patient-oriented research that has the potential to directly affect clinical practice. We use the term population-based research to refer to studies involving epidemiology, social and behavioral sciences, public health, quality evaluation, and cost-effectiveness.
In our model, the T1, T2, and T3 arrows represent bridges from one type of research to another. Examples of T1 research are drug development, pharmacogenomics, and some studies of disease mechanisms and research into new areas such as genetics, genomics, and proteomics. Examples of T2 are clinical epidemiology, health services (outcomes) research, and the newly developing methodology of community-based participatory research. Examples of T3 are emerging disciplines such as molecular and genetic epidemiology. T3 research highlights, for instance, how research in populations informs hypotheses that can be tested in basic science laboratories and how biomarkers in animal models can translate into population-based screening tools.
Implications for the design of training programs
The interaction of several disciplines is required to translate knowledge from one type of research to another (e.g., to move a basic science discovery to the bedside). Collaboration among disciplines through multidisciplinary teams facilitates the emergence of novel concepts and approaches to addressing important health issues. The emergence and development of new ideas are goals of translational research, and there are many possible models of training that can provide the academic path to these goals.
Training in translational research will vary depending on the background of trainees and the areas of research they plan to pursue. Given the diversity of educational backgrounds and research interests, it will be necessary to design a customized curriculum for almost every trainee. To ensure an understanding of complementary disciplines and to enhance communication and collaboration, trainees who have focused on basic laboratory research will need to become immersed in clinical sciences and clinical practice, while trainees with a clinical focus will need to gain exposure to basic science research. Both types of individuals will also benefit from training in population-based sciences, as is encouraged, for example, by the Burroughs Wellcome Fund, which sponsors the Institutional Program Unifying Population and Laboratory Based Sciences. 17
The details of a clinical immersion experience will depend on the area of research interest. For example, trainees interested in neuroscience may wish to accompany clinicians in a psychiatry or neurology clinic, and trainees working on bone tissue regeneration may participate in the activities of a clinical orthopedic surgery program. Trainees seeking laboratory immersion could take courses in techniques of molecular biology or genetics and work at the bench for a concentrated period of 3–4 months. Trainees who have a background in the social sciences or economics and are interested in health services research may need to join a team of investigators working in their area of interest.
All trainees could benefit from fundamental instruction concerning study design, data collection, statistical analysis, ethics and research integrity, protection of human subjects, the search for funding sources, the writing of institutional review board protocols and grant applications, the pursuit of patents and technology transfer, and government requirements for new drugs and devices. Because of the nature of translational research, it is also imperative for training programs to ensure that trainees develop the competencies needed to thrive in a multidisciplinary collaborative team. These competencies include communication and negotiation skills as well as ethical and humanitarian attitudes.
The most effective approach would be to design an individualized curriculum for each trainee, guided by a customized, learner-centered advisory committee that includes mentors with various and complementary backgrounds in clinical practice and basic and clinical research. One of the mentors would assume the role of primary mentor to ensure coordination of efforts and the success of the mentoring process.
Mentoring is a demanding but highly rewarding enterprise whose success depends on the widely varying skills, needs, and attitudes of different individuals. 18 Mentors who are able to monitor the incorporation and understanding of translational research essentials will be crucial to the positive outcomes of training programs. However, trainees will also need to have critical thinking skills and practical knowledge about how to work collaboratively and manage teams. Although most medical schools now realize the importance of teaching their students how to think critically, 19 the truth is, as Jerome Groopman points out, that the older generation of students were not taught to think as clinicians. 20 Although recent emphasis has shifted to training medical students and residents how to follow preset algorithms and decision trees, these approaches are challenging when clinicians need to think outside their domains. 20 Since clinical and translational research in this century necessitates out-of-the-box thinking, training programs must teach young researchers how to excel as critical thinkers.
Historically, medicine has taken a hands-off approach to teaching management and leadership, the notion being that learning how to manage and lead is simply intuitive. “Something about management looks so easy that we...never doubt that we could succeed where others repeatedly fail,” says Thomas Teal, former senior editor of the Harvard Business Review . 21 (pp3–4) Because managing is less a series of technical tasks and more a set of human interactions, managers and team leaders require what Daniel Goleman and his colleagues call “emotional intelligence” 22 and other skills that are not usually taught in research training programs. When we think of innovation and creative problem solving, we often look to engineers and designers to learn about these processes. 23 Similarly, when we think of management, we often look to the corporate sector. A useful approach to creating a supportive environment that fosters critical thinking and leadership and management skills must include the explicit training of fellows and junior faculty in these areas.
An effective training program in translational research must use traditional curricular elements in new ways to ensure understanding across disciplines. In addition, it must create and use new curricular elements and approaches to ensure that its trainees are able to do the following: critically examine the research process; think “out of the box” to develop ways to impact health care by transferring knowledge from and to the bench, bedside, and community; engage in multidisciplinary collaboration; understand successful approaches to community engagement; and develop appropriate techniques to manage multidisciplinary research teams in the future. Using multidisciplinary skills, the translational researcher will be able to think and perform in an integrated interdisciplinary manner and become a new type of investigator.
Meeting these goals is a challenge because research training programs are not traditionally content-based. We need to consider the creation of a community of learners and leaders by fostering the use of problem-based learning 24 , 25 as a gateway to collaborative leadership. Adopting these techniques will require a change in culture in medical schools, but the time is right to begin the process of this cultural shift if we wish to take a leap forward in enhancing the practice of moving from bench to bedside to community and back in translational research.
An approach to evaluation
With the necessity of customizing training in translational research, the approach to evaluation must be flexible. One of the most flexible approaches is to design a logic model that offers a graphic display of the relationships between program elements, objectives, and desired outcomes in the short term, intermediate term, and long term. The accompanying logic model ( List 1 ) provides an example of a framework for a training program in translational research. The logic model approach has the advantage of being adaptable as definitions of research and research goals evolve. Specific elements in the model can change, along with indicators and data sources, without completely disrupting the overall logical flow of objectives.
Logic Model for Training in Translational Research
In the logic model for a translational research program, the domains to be evaluated could include (1) whether the tools employed to achieve preestablished objectives, including general and scientific area specific competencies in translational sciences, are adequate; (2) whether the trainees acquire the cognitive and practical skills they need to effectively conduct translational research; (3) whether the trainees are successful in developing and pursuing a translational research career; and (4) whether the program as a whole promotes and enhances translational research. The outcomes of each of these domains could include (1) evidence that courses, seminars, workshops, and laboratory experiences offered in the program lead to fulfillment of preestablished competency requirements; (2) evidence of improvement over time in the trainees’ knowledge and skills regarding translational research topics and endeavors, as assessed via testing and via evaluations provided by scientific advisory committees; (3) evidence of successful career development, as measured by the ability to publish articles in peer-reviewed journals, to obtain research grants and academic appointments, and to gain leadership positions in multidisciplinary teams; and (4) evidence of the increased impact of the program at the institutional level and the national level, as judged by whether more translational research is funded and conducted at these levels.
We believe that translational research moves in a bidirectional manner from one type of research to another—from basic research to patient-oriented research, to population-based research, and back—and involves collaboration among scientists from multiple disciplines. The design of an effective training program in translational research is a challenge because the program must offer each of its trainees the opportunity to master a combination of skills that are not taught together in traditional training programs. The approach to evaluating the success of translational training programs must be flexible enough to accommodate the needs of individual institutions and individual trainees within the institutions, but it must also be rigorous enough to document that the program is meeting its short-, intermediate-, and long-term objectives and that its trainees are meeting preestablished competency requirements. A logic model framework with appropriate domains may be well suited to these evaluation efforts.
Acknowledgments
The authors wish to acknowledge the members of the Evaluation Committee of the Association for Clinical Research Training, especially Sunday Clark, ScD (University of Pittsburgh), Yvonne Belanger, MS (Duke University), David Goff MD, PhD (Wake Forest University), and Jan Hogle, PhD (University of Wisconsin).
Funding/Support: This research was supported by National Institutes of Health grants awarded to the University of Pittsburgh (UL1 RR024153), Duke University School of Medicine (UL1 RR024128), Albert Einstein College of Medicine (UL1 RR025750), Harvard Medical School (K30 RR022292), and University of Wisconsin (UL1 RR025011).
Other disclosures: None
Ethical approval: Not applicable
Contributor Information
Doris McGartland Rubio, Associate Professor and Director of the Data Center, Center for Research on Health Care, Division of General Internal Medicine, Department of Medicine, School of Medicine, University of Pittsburgh, Pittsburgh, PA.
Ellie E. Schoenbaum, Professor of Epidemiology & Population Health, Director Education and Career Development Core, Institute for Clinical and Translational Research, Institute for Clinical and Translational Research, Albert Einstein College of Medicine and Montefiore Medical Center, Bronx, NY.
Linda S. Lee, Associate Director for the Clinical Research Training Program, Department of Biostatistics and Bioinformatics, School of Medicine, Duke University, Durham, NC.
David E. Schteingart, Professor, Department of Internal Medicine, Division of Metabolism, Endocrinology and Diabetes; Co-Director, Education, Mentoring and Career Development Core, Michigan Institute for Clinical and Health Research; University of Michigan, Ann Arbor.
Paul R. Marantz, Associate Dean for Clinical Research Education and Professor, Department of Epidemiology and Population Health, Albert Einstein College of Medicine and Montefiore Medical Center, Bronx, NY.
Karl E. Anderson, Professor, Department of Preventive Medicine and Community Health, University of Texas Medical Branch, Galveston, TX.
Lauren Dewey Platt, Executive Director, Scholars in Clinical Science Program, Harvard Catalyst/The Harvard Clinical and Translational Science Center, Harvard Medical School, Tosteson Medical Education Center, Boston, MA.
Adriana Baez, Professor, Departments of Pharmacology and Otolaryngology-Head and Neck Surgery, University of Puerto Rico School of Medicine, San Juan, Puerto Rico.
Karin Esposito, Assistant Professor of Clinical Psychiatry, Department of Psychiatry and Behavioral Sciences, Miller School of Medicine, University of Miami, Miami, FL.

Drs. Goyal and Tarini to lead Center for Translational Research
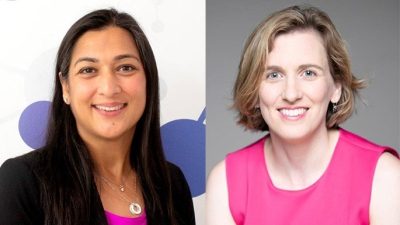
As CTR co-directors, Drs. Goyal and Tarini will lead the hospital’s mission to advance translational science, clinical research and community health.
Children’s National Hospital has appointed two nationally regarded leaders in pediatric research – Monika K. Goyal, M.D., M.S.C.E. , and Beth A. Tarini, M.D., M.S., M.B.A. – to head its Center for Translational Research (CTR), a hub of high-impact scientific investigation that touches nearly every pediatric specialty.
As CTR co-directors, Drs. Goyal and Tarini will lead the hospital’s mission to advance translational science, clinical research and community health. They will begin their new roles on July 1.
Moving the field forward
“It is truly an honor to lead the CTR at such a pivotal moment in pediatric health,” said Dr. Goyal, an emergency medicine specialist and health services researcher. “I look forward to helping Children’s National lead the science on advancing health equity for the patients, families and communities we serve, both locally and nationally.”
As the largest of the six centers within the Children’s National Research Institute , CTR is pivotal in finding groundbreaking ways to improve health across pediatric medicine. Using a “bench to bedside” approach, the CTR faculty strives to seamlessly translate science from the laboratory bench to the patient’s bedside, moving pediatric medicine forward as expeditiously as possible to bring advances into the community.
“CTR is uniquely positioned to solve the biggest healthcare issues facing our pediatric patients,” said Dr. Tarini, a pediatrician and national leader in newborn screening research and policy. “I look forward to leading our diverse faculty of physicians and researchers as they leverage their front-line experience and innovative research to improve child health.”
Why we’re excited
Dr. Tarini joined Children’s National in 2018 and is currently the associate director for CTR. She was recently promoted to tenured professor of Pediatrics at George Washington University and has extensively studied policies to optimize the delivery of genetic services to families of newborns. In January, Dr. Tarini was appointed to a National Academies of Sciences, Engineering and Medicine Committee to examine the current landscape of newborn screening systems, processes and research in the United States. Dr. Tarini has obtained $10 million in federal and foundation funding, and she has served as president of the Society for Pediatric Research.
Dr. Goyal joined Children’s National in 2012. She is the inaugural endowed chair for Women in Science and Health and has served as the associate division chief for Academic Affairs and Research within the Emergency Department since 2018. She was recently promoted to tenured professor of Pediatrics and Emergency Medicine at George Washington University. Dr. Goyal is a nationally renowned equity science scholar and has published over 130 peer-reviewed manuscripts. She has secured more than $25 million in federal and foundation funding to address disparities in adolescent sexual health, pain management and firearm violence.
Children’s National leads the way
Catherine Bollard, M.D., M.B.Ch.B. , interim chief academic officer, said she looks forward to seeing the advances in pediatric health guided by these two outstanding researchers. “By harnessing the immense talent within Children’s National for our search, we found two exceptional leaders in Drs. Goyal and Tarini,” Dr. Bollard said. “Their work promoting research that accelerates discovery across the continuum of bench, bedside and community has already made a significant impact.”
You might also like
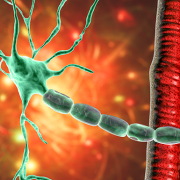
- Children’s National Research Institute
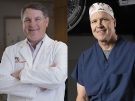
- Download PDF
- CME & MOC
- Share X Facebook Email LinkedIn
- Permissions
Accounting for Competing Risks in Clinical Research
- 1 Institute for Clinical Evaluative Sciences (ICES), Toronto, Ontario, Canada
- 2 Institute of Health Policy, Management and Evaluation, University of Toronto, Toronto, Ontario, Canada
- 3 Sunnybrook Research Institute, Toronto, Ontario, Canada
- 4 NHS Blood and Transplant, Bristol, United Kingdom
- 5 Department of Biomedical Data Sciences, Leiden University Medical Center, Leiden, the Netherlands
- Original Investigation Metformin or Sulfonylurea Use in Kidney Disease Christianne L. Roumie, MD, MPH; Jonathan Chipman, PhD; Jea Young Min, PharmD, MPH, PhD; Amber J. Hackstadt, PhD; Adriana M. Hung, MD, MPH; Robert A. Greevy Jr, PhD; Carlos G. Grijalva, MD, MPH; Tom Elasy, MD, MPH; Marie R. Griffin, MD, MPH JAMA
Survival analyses are statistical methods for the analysis of time-to-event outcomes. 1 An example is time from study entry to death. A competing risk is an event whose occurrence precludes the occurrence of the primary event of interest. In a study whose outcome is time to death due to cardiovascular causes, for instance, death due to a noncardiovascular cause is a competing risk. Conventional statistical methods for the analysis of survival data typically aim to estimate the probability of the event of interest over time or the effect of a risk factor or treatment on that probability or on the intensity with which events occur. These methods require modification in the presence of competing risks. A key feature of survival analysis is the ability to properly account for censoring, which occurs when the outcome event is not observed before the end of the study participant’s follow-up period.
Read More About
Austin PC , Ibrahim M , Putter H. Accounting for Competing Risks in Clinical Research. JAMA. Published online May 29, 2024. doi:10.1001/jama.2024.4970
Manage citations:
© 2024
Artificial Intelligence Resource Center
Cardiology in JAMA : Read the Latest
Browse and subscribe to JAMA Network podcasts!
Others Also Liked
Select your interests.
Customize your JAMA Network experience by selecting one or more topics from the list below.
- Academic Medicine
- Acid Base, Electrolytes, Fluids
- Allergy and Clinical Immunology
- American Indian or Alaska Natives
- Anesthesiology
- Anticoagulation
- Art and Images in Psychiatry
- Artificial Intelligence
- Assisted Reproduction
- Bleeding and Transfusion
- Caring for the Critically Ill Patient
- Challenges in Clinical Electrocardiography
- Climate and Health
- Climate Change
- Clinical Challenge
- Clinical Decision Support
- Clinical Implications of Basic Neuroscience
- Clinical Pharmacy and Pharmacology
- Complementary and Alternative Medicine
- Consensus Statements
- Coronavirus (COVID-19)
- Critical Care Medicine
- Cultural Competency
- Dental Medicine
- Dermatology
- Diabetes and Endocrinology
- Diagnostic Test Interpretation
- Drug Development
- Electronic Health Records
- Emergency Medicine
- End of Life, Hospice, Palliative Care
- Environmental Health
- Equity, Diversity, and Inclusion
- Facial Plastic Surgery
- Gastroenterology and Hepatology
- Genetics and Genomics
- Genomics and Precision Health
- Global Health
- Guide to Statistics and Methods
- Hair Disorders
- Health Care Delivery Models
- Health Care Economics, Insurance, Payment
- Health Care Quality
- Health Care Reform
- Health Care Safety
- Health Care Workforce
- Health Disparities
- Health Inequities
- Health Policy
- Health Systems Science
- History of Medicine
- Hypertension
- Images in Neurology
- Implementation Science
- Infectious Diseases
- Innovations in Health Care Delivery
- JAMA Infographic
- Law and Medicine
- Leading Change
- Less is More
- LGBTQIA Medicine
- Lifestyle Behaviors
- Medical Coding
- Medical Devices and Equipment
- Medical Education
- Medical Education and Training
- Medical Journals and Publishing
- Mobile Health and Telemedicine
- Narrative Medicine
- Neuroscience and Psychiatry
- Notable Notes
- Nutrition, Obesity, Exercise
- Obstetrics and Gynecology
- Occupational Health
- Ophthalmology
- Orthopedics
- Otolaryngology
- Pain Medicine
- Palliative Care
- Pathology and Laboratory Medicine
- Patient Care
- Patient Information
- Performance Improvement
- Performance Measures
- Perioperative Care and Consultation
- Pharmacoeconomics
- Pharmacoepidemiology
- Pharmacogenetics
- Pharmacy and Clinical Pharmacology
- Physical Medicine and Rehabilitation
- Physical Therapy
- Physician Leadership
- Population Health
- Primary Care
- Professional Well-being
- Professionalism
- Psychiatry and Behavioral Health
- Public Health
- Pulmonary Medicine
- Regulatory Agencies
- Reproductive Health
- Research, Methods, Statistics
- Resuscitation
- Rheumatology
- Risk Management
- Scientific Discovery and the Future of Medicine
- Shared Decision Making and Communication
- Sleep Medicine
- Sports Medicine
- Stem Cell Transplantation
- Substance Use and Addiction Medicine
- Surgical Innovation
- Surgical Pearls
- Teachable Moment
- Technology and Finance
- The Art of JAMA
- The Arts and Medicine
- The Rational Clinical Examination
- Tobacco and e-Cigarettes
- Translational Medicine
- Trauma and Injury
- Treatment Adherence
- Ultrasonography
- Users' Guide to the Medical Literature
- Vaccination
- Venous Thromboembolism
- Veterans Health
- Women's Health
- Workflow and Process
- Wound Care, Infection, Healing
- Register for email alerts with links to free full-text articles
- Access PDFs of free articles
- Manage your interests
- Save searches and receive search alerts
- About the Hub
- Announcements
- Faculty Experts Guide
- Subscribe to the newsletter
Explore by Topic
- Arts+Culture
- Politics+Society
- Science+Technology
- Student Life
- University News
- Voices+Opinion
- About Hub at Work
- Gazette Archive
- Benefits+Perks
- Health+Well-Being
- Current Issue
- About the Magazine
- Past Issues
- Support Johns Hopkins Magazine
- Subscribe to the Magazine
You are using an outdated browser. Please upgrade your browser to improve your experience.
Elizabeth Selvin named director of the Welch Center for Epidemiology and Clinical Research
Selvin has devoted her career to leading translational research projects designed to evaluate and improve screening, diagnosis, and patient care for persons with diabetes.
By Hub staff report
Elizabeth Selvin has been appointed the next director of the Welch Center for Epidemiology and Clinical Research at the Johns Hopkins University, effective May 1. She succeeds Lawrence J. Appel, who led the center for the past 14 years.
A collaborative effort between the Johns Hopkins Bloomberg School of Public Health , the School of Medicine , and the School of Nursing , the Welch Center was established in 1989 with the primary mission of improving population health by conducting rigorous research that integrates clinical medicine with public health expertise and by training the next generation of interdisciplinary researchers and scholars.
Image caption: Elizabeth Selvin
An internationally recognized leader in the field, Selvin is a professor of Epidemiology at the Bloomberg School and holds a joint appointment in the School of Medicine, Division of General Internal Medicine. She is the author or co-author of over 550 peer-reviewed papers. Selvin, who earned her PhD in public health from the Bloomberg School in 2004, has devoted her career to leading translational research projects designed to evaluate and improve screening, diagnosis, and patient care for persons with diabetes. Her work has directly influenced clinical practice guidelines for diagnosis and management of diabetes. Her many awards include the Harry Keen Memorial Award from the International Diabetes Epidemiology Group of the International Diabetes Federation and the Kelly West Award for Outstanding Achievement in Epidemiology from the American Diabetes Association. Selvin currently serves as a deputy editor of Diabetes Care .
In an announcement to the Hopkins community, leaders of the three schools collaborating at the Welch Center expressed gratitude for Appel's extraordinary leadership and congratulated Selvin for her accomplishments, noting her ongoing commitment to training the next generation of students and fellows from across the university. In 2024, she received the David M. Levine Excellence in Mentoring Award from the Johns Hopkins Department of Medicine.
"For over three decades, the Welch Center has fostered the integration of public health, medical, and nursing expertise to tackle some of the leading causes of mortality and morbidity here at home and globally," wrote School of Medicine Dean and CEO of Johns Hopkins Medicine Theodore L. DeWeese , Bloomberg School Dean Ellen J. MacKenzie , and School of Nursing Dean Sarah L. Szanton . "It is widely recognized as one of the world's premier centers for training and research in clinical epidemiology, with a focus on translating science for impact. We look forward to the next chapter in the center's remarkable history under Dr. Selvin's leadership."
Posted in University News
Tagged interdisciplinary research , johns hopkins medicine , school of public health , school of nursing
You might also like
News network.
- Johns Hopkins Magazine
- Get Email Updates
- Submit an Announcement
- Submit an Event
- Privacy Statement
- Accessibility
Discover JHU
- About the University
- Schools & Divisions
- Academic Programs
- Plan a Visit
- my.JohnsHopkins.edu
- © 2024 Johns Hopkins University . All rights reserved.
- University Communications
- 3910 Keswick Rd., Suite N2600, Baltimore, MD
- X Facebook LinkedIn YouTube Instagram
- Open access
- Published: 27 May 2024
Current status of community resources and priorities for weed genomics research
- Jacob Montgomery 1 ,
- Sarah Morran 1 ,
- Dana R. MacGregor ORCID: orcid.org/0000-0003-0543-0408 2 ,
- J. Scott McElroy ORCID: orcid.org/0000-0003-0331-3697 3 ,
- Paul Neve ORCID: orcid.org/0000-0002-3136-5286 4 ,
- Célia Neto ORCID: orcid.org/0000-0003-3256-5228 4 ,
- Martin M. Vila-Aiub ORCID: orcid.org/0000-0003-2118-290X 5 ,
- Maria Victoria Sandoval 5 ,
- Analia I. Menéndez ORCID: orcid.org/0000-0002-9681-0280 6 ,
- Julia M. Kreiner ORCID: orcid.org/0000-0002-8593-1394 7 ,
- Longjiang Fan ORCID: orcid.org/0000-0003-4846-0500 8 ,
- Ana L. Caicedo ORCID: orcid.org/0000-0002-0378-6374 9 ,
- Peter J. Maughan 10 ,
- Bianca Assis Barbosa Martins 11 ,
- Jagoda Mika 11 ,
- Alberto Collavo 11 ,
- Aldo Merotto Jr. ORCID: orcid.org/0000-0002-1581-0669 12 ,
- Nithya K. Subramanian ORCID: orcid.org/0000-0002-1659-7396 13 ,
- Muthukumar V. Bagavathiannan ORCID: orcid.org/0000-0002-1107-7148 13 ,
- Luan Cutti ORCID: orcid.org/0000-0002-2867-7158 14 ,
- Md. Mazharul Islam 15 ,
- Bikram S. Gill ORCID: orcid.org/0000-0003-4510-9459 16 ,
- Robert Cicchillo 17 ,
- Roger Gast 17 ,
- Neeta Soni ORCID: orcid.org/0000-0002-4647-8355 17 ,
- Terry R. Wright ORCID: orcid.org/0000-0002-3969-2812 18 ,
- Gina Zastrow-Hayes 18 ,
- Gregory May 18 ,
- Jenna M. Malone ORCID: orcid.org/0000-0002-9637-2073 19 ,
- Deepmala Sehgal ORCID: orcid.org/0000-0002-4141-1784 20 ,
- Shiv Shankhar Kaundun ORCID: orcid.org/0000-0002-7249-2046 20 ,
- Richard P. Dale 20 ,
- Barend Juan Vorster ORCID: orcid.org/0000-0003-3518-3508 21 ,
- Bodo Peters 11 ,
- Jens Lerchl ORCID: orcid.org/0000-0002-9633-2653 22 ,
- Patrick J. Tranel ORCID: orcid.org/0000-0003-0666-4564 23 ,
- Roland Beffa ORCID: orcid.org/0000-0003-3109-388X 24 ,
- Alexandre Fournier-Level ORCID: orcid.org/0000-0002-6047-7164 25 ,
- Mithila Jugulam ORCID: orcid.org/0000-0003-2065-9067 15 ,
- Kevin Fengler 18 ,
- Victor Llaca ORCID: orcid.org/0000-0003-4822-2924 18 ,
- Eric L. Patterson ORCID: orcid.org/0000-0001-7111-6287 14 &
- Todd A. Gaines ORCID: orcid.org/0000-0003-1485-7665 1
Genome Biology volume 25 , Article number: 139 ( 2024 ) Cite this article
194 Accesses
1 Altmetric
Metrics details
Weeds are attractive models for basic and applied research due to their impacts on agricultural systems and capacity to swiftly adapt in response to anthropogenic selection pressures. Currently, a lack of genomic information precludes research to elucidate the genetic basis of rapid adaptation for important traits like herbicide resistance and stress tolerance and the effect of evolutionary mechanisms on wild populations. The International Weed Genomics Consortium is a collaborative group of scientists focused on developing genomic resources to impact research into sustainable, effective weed control methods and to provide insights about stress tolerance and adaptation to assist crop breeding.
Each year globally, agricultural producers and landscape managers spend billions of US dollars [ 1 , 2 ] and countless hours attempting to control weedy plants and reduce their adverse effects. These management methods range from low-tech (e.g., pulling plants from the soil by hand) to extremely high-tech (e.g., computer vision-controlled spraying of herbicides). Regardless of technology level, effective control methods serve as strong selection pressures on weedy plants and often result in rapid evolution of weed populations resistant to such methods [ 3 , 4 , 5 , 6 , 7 ]. Thus, humans and weeds have been locked in an arms race, where humans develop new or improved control methods and weeds adapt and evolve to circumvent such methods.
Applying genomics to weed science offers a unique opportunity to study rapid adaptation, epigenetic responses, and examples of evolutionary rescue of diverse weedy species in the face of widespread and powerful selective pressures. Furthermore, lessons learned from these studies may also help to develop more sustainable control methods and to improve crop breeding efforts in the face of our ever-changing climate. While other research fields have used genetics and genomics to uncover the basis of many biological traits [ 8 , 9 , 10 , 11 ] and to understand how ecological factors affect evolution [ 12 , 13 ], the field of weed science has lagged behind in the development of genomic tools essential for such studies [ 14 ]. As research in human and crop genetics pushes into the era of pangenomics (i.e., multiple chromosome scale genome assemblies for a single species [ 15 , 16 ]), publicly available genomic information is still lacking or severely limited for the majority of weed species. Recent reviews of current weed genomes identified 26 [ 17 ] and 32 weed species with sequenced genomes [ 18 ]—many assembled to a sub-chromosome level.
Here, we summarize the current state of weed genomics, highlighting cases where genomics approaches have successfully provided insights on topics such as population genetic dynamics, genome evolution, and the genetic basis of herbicide resistance, rapid adaptation, and crop dedomestication. These highlighted investigations all relied upon genomic resources that are relatively rare for weedy species. Throughout, we identify additional resources that would advance the field of weed science and enable further progress in weed genomics. We then introduce the International Weed Genomics Consortium (IWGC), an open collaboration among researchers, and describe current efforts to generate these additional resources.
Evolution of weediness: potential research utilizing weed genomics tools
Weeds can evolve from non-weed progenitors through wild colonization, crop de-domestication, or crop-wild hybridization [ 19 ]. Because the time span in which weeds have evolved is necessarily limited by the origins of agriculture, these non-weed relatives often still exist and can be leveraged through population genomic and comparative genomic approaches to identify the adaptive changes that have driven the evolution of weediness. The ability to rapidly adapt, persist, and spread in agroecosystems are defining features of weedy plants, leading many to advocate agricultural weeds as ideal candidates for studying rapid plant adaptation [ 20 , 21 , 22 , 23 ]. The insights gained from applying plant ecological approaches to the study of rapid weed adaptation will move us towards the ultimate goals of mitigating such adaptation and increasing the efficacy of crop breeding and biotechnology [ 14 ].
Biology and ecological genomics of weeds
The impressive community effort to create and maintain resources for Arabidopsis thaliana ecological genomics provides a motivating example for the emerging study of weed genomics [ 24 , 25 , 26 , 27 ]. Arabidopsis thaliana was the first flowering plant species to have its genome fully sequenced [ 28 ] and rapidly became a model organism for plant molecular biology. As weedy genomes become available, collection, maintenance, and resequencing of globally distributed accessions of these species will help to replicate the success found in ecological studies of A. thaliana [ 29 , 30 , 31 , 32 , 33 , 34 , 35 ]. Evaluation of these accessions for traits of interest to produce large phenomics data sets (as in [ 36 , 37 , 38 , 39 , 40 ]) enables genome-wide association studies and population genomics analyses aimed at dissecting the genetic basis of variation in such traits [ 41 ]. Increasingly, these resources (e.g. the 1001 genomes project [ 29 ]) have enabled A. thaliana to be utilized as a model species to explore the eco-evolutionary basis of plant adaptation in a more realistic ecological context. Weedy species should supplement lessons in eco-evolutionary genomics learned from these experiments in A. thaliana .
Untargeted genomic approaches for understanding the evolutionary trajectories of populations and the genetic basis of traits as described above rely on the collection of genotypic information from across the genome of many individuals. While whole-genome resequencing accomplishes this requirement and requires no custom methodology, this approach provides more information than is necessary and is prohibitively expensive in species with large genomes. Development and optimization of genotype-by-sequencing methods for capturing reduced representations of newly sequence genomes like those described by [ 42 , 43 , 44 ] will reduce the cost and computational requirements of genetic mapping and population genetic experiments. Most major weed species do not currently have protocols for stable transformation, a key development in the popularity of A. thaliana as a model organism and a requirement for many functional genomic approaches. Functional validation of genes/variants believed to be responsible for traits of interest in weeds has thus far relied on transiently manipulating endogenous gene expression [ 45 , 46 ] or ectopic expression of a transgene in a model system [ 47 , 48 , 49 ]. While these methods have been successful, few weed species have well-studied viral vectors to adapt for use in virus induced gene silencing. Spray induced gene silencing is another potential option for functional investigation of candidate genes in weeds, but more research is needed to establish reliable delivery and gene knockdown [ 50 ]. Furthermore, traits with complex genetic architecture divergent between the researched and model species may not be amenable to functional genomic approaches using transgenesis techniques in model systems. Developing protocols for reduced representation sequencing, stable transformation, and gene editing/silencing in weeds will allow for more thorough characterization of candidate genetic variants underlying traits of interest.
Beyond rapid adaptation, some weedy species offer an opportunity to better understand co-evolution, like that between plants and pollinators and how their interaction leads to the spread of weedy alleles (Additional File 1 : Table S1). A suite of plant–insect traits has co-evolved to maximize the attraction of the insect pollinator community and the efficiency of pollen deposition between flowers ensuring fruit and seed production in many weeds [ 51 , 52 ]. Genetic mapping experiments have identified genes and genetic variants responsible for many floral traits affecting pollinator interaction including petal color [ 53 , 54 , 55 , 56 ], flower symmetry and size [ 57 , 58 , 59 ], and production of volatile organic compounds [ 60 , 61 , 62 ] and nectar [ 63 , 64 , 65 ]. While these studies reveal candidate genes for selection under co-evolution, herbicide resistance alleles may also have pleiotropic effects on the ecology of weeds [ 66 ], altering plant-pollinator interactions [ 67 ]. Discovery of genes and genetic variants involved in weed-pollinator interaction and their molecular and environmental control may create opportunities for better management of weeds with insect-mediated pollination. For example, if management can disrupt pollinator attraction/interaction with these weeds, the efficiency of reproduction may be reduced.
A more complete understanding of weed ecological genomics will undoubtedly elucidate many unresolved questions regarding the genetic basis of various aspects of weediness. For instance, when comparing populations of a species from agricultural and non-agricultural environments, is there evidence for contemporary evolution of weedy traits selected by agricultural management or were “natural” populations pre-adapted to agroecosystems? Where there is differentiation between weedy and natural populations, which traits are under selection and what is the genetic basis of variation in those traits? When comparing between weedy populations, is there evidence for parallel versus non-parallel evolution of weediness at the phenotypic and genotypic levels? Such studies may uncover fundamental truths about weediness. For example, is there a common phenotypic and/or genotypic basis for aspects of weediness among diverse weed species? The availability of characterized accessions and reference genomes for species of interest are required for such studies but only a few weedy species have these resources developed.
Population genomics
Weed species are certainly fierce competitors, able to outcompete crops and endemic species in their native environment, but they are also remarkable colonizers of perturbed habitats. Weeds achieve this through high fecundity, often producing tens of thousands of seeds per individual plant [ 68 , 69 , 70 ]. These large numbers in terms of demographic population size often combine with outcrossing reproduction to generate high levels of diversity with local effective population sizes in the hundreds of thousands [ 71 , 72 ]. This has two important consequences: weed populations retain standing genetic variation and generate many new mutations, supporting weed success in the face of harsh control. The generation of genomic tools to monitor weed populations at the molecular level is a game-changer to understanding weed dynamics and precisely testing the effect of artificial selection (i.e., management) and other evolutionary mechanisms on the genetic make-up of populations.
Population genomic data, without any environmental or phenotypic information, can be used to scan the genomes of weed and non-weed relatives to identify selective sweeps, pointing at loci supporting weed adaptation on micro- or macro-evolutionary scales. Two recent within-species examples include weedy rice, where population differentiation between weedy and domesticated populations was used to identify the genetic basis of weedy de-domestication [ 73 ], and common waterhemp, where consistent allelic differences among natural and agricultural collections resolved a complex set of agriculturally adaptive alleles [ 74 , 75 ]. A recent comparative population genomic study of weedy barnyardgrass and crop millet species has demonstrated how inter-specific investigations can resolve the signatures of crop and weed evolution [ 76 ] (also see [ 77 ] for a non-weed climate adaptation example). Multiple sequence alignments across numerous species provide complementary insight into adaptive convergence over deeper timescales, even with just one genomic sample per species (e.g., [ 78 , 79 ]). Thus, newly sequenced weed genomes combined with genomes available for closely related crops (outlined by [ 14 , 80 ]) and an effort to identify other non-weed wild relatives will be invaluable in characterizing the genetic architecture of weed adaptation and evolution across diverse species.
Weeds experience high levels of genetic selection, both artificial in response to agricultural practices and particularly herbicides, and natural in response to the environmental conditions they encounter [ 81 , 82 ]. Using genomic analysis to identify loci that are the targets of selection, whether natural or artificial, would point at vulnerabilities that could be leveraged against weeds to develop new and more sustainable management strategies [ 83 ]. This is a key motivation to develop genotype-by-environment association (GEA) and selective sweep scan approaches, which allow researchers to resolve the molecular basis of multi-dimensional adaptation [ 84 , 85 ]. GEA approaches, in particular, have been widely used on landscape-wide resequencing collections to determine the genetic basis of climate adaptation (e.g., [ 27 , 86 , 87 ]), but have yet to be fully exploited to diagnose the genetic basis of the various aspects of weediness [ 88 ]. Armed with data on environmental dimensions of agricultural settings, such as focal crop, soil quality, herbicide use, and climate, GEA approaches can help disentangle how discrete farming practices have influenced the evolution of weediness and resolve broader patterns of local adaptation across a weed’s range. Although non-weedy relatives are not technically required for GEA analyses, inclusion of environmental and genomic data from weed progenitors can further distinguish genetic variants underpinning weed origins from those involved in local adaptation.
New weeds emerge frequently [ 89 ], either through hybridization between species as documented for sea beet ( Beta vulgaris ssp. maritima) hybridizing with crop beet to produce progeny that are well adapted to agricultural conditions [ 90 , 91 , 92 ], or through the invasion of alien species that find a new range to colonize. Biosecurity measures are often in place to stop the introduction of new weeds; however, the vast scale of global agricultural commodity trade precludes the possibility of total control. Population genomic analysis is now able to measure gene flow between populations [ 74 , 93 , 94 , 95 ] and identify populations of origin for invasive species including weeds [ 96 , 97 , 98 ]. For example, the invasion route of the pest fruitfly Drosophila suzukii from Eastern Asia to North America and Europe through Hawaii was deciphered using Approximate Bayesian Computation on high-throughput sequencing data from a global sample of multiple populations [ 99 ]. Genomics can also be leveraged to predict invasion rather than explain it. The resequencing of a global sample of common ragweed ( Ambrosia artemisiifolia L.) elucidated a complex invasion route whereby Europe was invaded by multiple introductions of American ragweed that hybridized in Europe prior to a subsequent introduction to Australia [ 100 , 101 ]. In this context, the use of genomically informed species distribution models helps assess the risk associated with different source populations, which in the case of common ragweed, suggests that a source population from Florida would allow ragweed to invade most of northern Australia [ 102 ]. Globally coordinated research efforts to understand potential distribution models could support the transformation of biosecurity from perspective analysis towards predictive risk assessment.
Herbicide resistance and weed management
Herbicide resistance is among the numerous weedy traits that can evolve in plant populations exposed to agricultural selection pressures. Over-reliance on herbicides to control weeds, along with low diversity and lack of redundancy in weed management strategies, has resulted in globally widespread herbicide resistance [ 103 ]. To date, 272 herbicide-resistant weed species have been reported worldwide, and at least one resistance case exists for 21 of the 31 existing herbicide sites of action [ 104 ]—significantly limiting chemical weed control options available to agriculturalists. This limitation of control options is exacerbated by the recent lack of discovery of herbicides with new sites of action [ 105 ].
Herbicide resistance may result from several different physiological mechanisms. Such mechanisms have been classified into two main groups, target-site resistance (TSR) [ 4 , 106 ] and non-target-site resistance (NTSR) [ 4 , 107 ]. The first group encompasses changes that reduce binding affinity between a herbicide and its target [ 108 ]. These changes may provide resistance to multiple herbicides that have a common biochemical target [ 109 ] and can be effectively managed through mixture and/or rotation of herbicides targeting different sites of action [ 110 ]. The second group (NTSR), includes alterations in herbicide absorption, translocation, sequestration, and/or metabolism that may lead to unpredictable pleotropic cross-resistance profiles where structurally and functionally diverse herbicides are rendered ineffective by one or more genetic variant(s) [ 47 ]. This mechanism of resistance threatens not only the efficacy of existing herbicidal chemistries, but also ones yet to be discovered. While TSR is well understood because of the ease of identification and molecular characterization of target site variants, NTSR mechanisms are significantly more challenging to research because they are often polygenic, and the resistance causing element(s) are not well understood [ 111 ].
Improving the current understanding of metabolic NTSR mechanisms is not an easy task, since genes of diverse biochemical functions are involved, many of which exist as extensive gene families [ 109 , 112 ]. Expression changes of NTSR genes have been implicated in several resistance cases where the protein products of the genes are functionally equivalent across sensitive and resistant plants, but their relative abundance leads to resistance. Thus, regulatory elements of NTSR genes have been scrutinized to understand their role in NTSR mechanisms [ 113 ]. Similarly, epigenetic modifications have been hypothesized to play a role in NTSR, with much remaining to be explored [ 114 , 115 , 116 ]. Untargeted approaches such as genome-wide association, selective sweep scans, linkage mapping, RNA-sequencing, and metabolomic profiling have proven helpful to complement more specific biochemical- and chemo-characterization studies towards the elucidation of NTSR mechanisms as well as their regulation and evolution [ 47 , 117 , 118 , 119 , 120 , 121 , 122 , 123 , 124 ]. Even in cases where resistance has been attributed to TSR, genetic mapping approaches can detect other NTSR loci contributing to resistance (as shown by [ 123 ]) and provide further evidence for the role of TSR mutations across populations. Knowledge of the genetic basis of NTSR will aid the rational design of herbicides by screening new compounds for interaction with newly discovered NTSR proteins during early research phases and by identifying conserved chemical structures that interact with these proteins that should be avoided in small molecule design.
Genomic resources can also be used to predict the protein structure for novel herbicide target site and metabolism genes. This will allow for prediction of efficacy and selectivity for new candidate herbicides in silico to increase herbicide discovery throughput as well as aid in the design and development of next-generation technologies for sustainable weed management. Proteolysis targeting chimeras (PROTACs) have the potential to bind desired targets with great selectivity and degrade proteins by utilizing natural protein ubiquitination and degradation pathways within plants [ 125 ]. Spray-induced gene silencing in weeds using oligonucleotides has potential as a new, innovative, and sustainable method for weed management, but improved methods for design and delivery of oligonucleotides are needed to make this technique a viable management option [ 50 ]. Additionally, success in the field of pharmaceutical drug discovery in the development of molecules modulating protein–protein interactions offers another potential avenue towards the development of herbicides with novel targets [ 126 , 127 ]. High-quality reference genomes allow for the design of new weed management technologies like the ones listed here that are specific to—and effective across—weed species but have a null effect on non-target organisms.
Comparative genomics and genome biology
The genomes of weed species are as diverse as weed species themselves. Weeds are found across highly diverged plant families and often have no phylogenetically close model or crop species relatives for comparison. On all measurable metrics, weed genomes run the gamut. Some have smaller genomes like Cyperus spp. (~ 0.26 Gb) while others are larger, such as Avena fatua (~ 11.1 Gb) (Table 1 ). Some have high heterozygosity in terms of single-nucleotide polymorphisms, such as the Amaranthus spp., while others are primarily self-pollinated and quite homozygous, such as Poa annua [ 128 , 129 ]. Some are diploid such as Conyza canadensis and Echinochloa haploclada while others are polyploid such as C. sumetrensis , E. crus-galli , and E. colona [ 76 ]. The availability of genomic resources in these diverse, unexplored branches of the tree of life allows us to identify consistencies and anomalies in the field of genome biology.
The weed genomes published so far have focused mainly on weeds of agronomic crops, and studies have revolved around their ability to resist key herbicides. For example, genomic resources were vital in the elucidation of herbicide resistance cases involving target site gene copy number variants (CNVs). Gene CNVs of 5-enolpyruvylshikimate-3-phosphate synthase ( EPSPS ) have been found to confer resistance to the herbicide glyphosate in diverse weed species. To date, nine species have independently evolved EPSPS CNVs, and species achieve increased EPSPS copy number via different mechanisms [ 153 ]. For instance, the EPSPS CNV in Bassia scoparia is caused by tandem duplication, which is accredited to transposable element insertions flanking EPSPS and subsequent unequal crossing over events [ 154 , 155 ]. In Eleusine indica , a EPSPS CNV was caused by translocation of the EPSPS locus into the subtelomere followed by telomeric sequence exchange [ 156 ]. One of the most fascinating genome biology discoveries in weed science has been that of extra-chromosomal circular DNAs (eccDNAs) that harbor the EPSPS gene in the weed species Amaranthus palmeri [ 157 , 158 ]. In this case, the eccDNAs autonomously replicate separately from the nuclear genome and do not reintegrate into chromosomes, which has implications for inheritance, fitness, and genome structure [ 159 ]. These discoveries would not have been possible without reference assemblies of weed genomes, next-generation sequencing, and collaboration with experts in plant genomics and bioinformatics.
Another question that is often explored with weedy genomes is the nature and composition of gene families that are associated with NTSR. Gene families under consideration often include cytochrome P450s (CYPs), glutathione- S -transferases (GSTs), ABC transporters, etc. Some questions commonly considered with new weed genomes include how many genes are in each of these gene families, where are they located, and which weed accessions and species have an over-abundance of them that might explain their ability to evolve resistance so rapidly [ 76 , 146 , 160 , 161 ]? Weed genome resources are necessary to answer questions about gene family expansion or contraction during the evolution of weediness, including the role of polyploidy in NTSR gene family expansion as explored by [ 162 ].
Translational research and communication with weed management stakeholders
Whereas genomics of model plants is typically aimed at addressing fundamental questions in plant biology, and genomics of crop species has the obvious goal of crop improvement, goals of genomics of weedy plants also include the development of more effective and sustainable strategies for their management. Weed genomic resources assist with these objectives by providing novel molecular ecological and evolutionary insights from the context of intensive anthropogenic management (which is lacking in model plants), and offer knowledge and resources for trait discovery for crop improvement, especially given that many wild crop relatives are also important agronomic weeds (e.g., [ 163 ]). For instance, crop-wild relatives are valuable for improving crop breeding for marginal environments [ 164 ]. Thus, weed genomics presents unique opportunities and challenges relative to plant genomics more broadly. It should also be noted that although weed science at its core is an applied discipline, it draws broadly from many scientific disciplines such as, plant physiology, chemistry, ecology, and evolutionary biology, to name a few. The successful integration of weed-management strategies, therefore, requires extensive collaboration among individuals collectively possessing the necessary expertise [ 165 ].
With the growing complexity of herbicide resistance management, practitioners are beginning to recognize the importance of understanding resistance mechanisms to inform appropriate management tactics [ 14 ]. Although weed science practitioners do not need to understand the technical details of weed genomics, their appreciation of the power of weed genomics—together with their unique insights from field observations—will yield novel opportunities for applications of weed genomics to weed management. In particular, combining field management history with information on weed resistance mechanisms is expected to provide novel insights into evolutionary trajectories (e.g. [ 6 , 166 ]), which can be utilized for disrupting evolutionary adaptation. It can be difficult to obtain field history information from practitioners, but developing an understanding among them of the importance of such information can be invaluable.
Development of weed genomics resources by the IWGC
Weed genomics is a fast-growing field of research with many recent breakthroughs and many unexplored areas of study. The International Weed Genomics Consortium (IWGC) started in 2021 to address the roadblocks listed above and to promote the study of weedy plants. The IWGC is an open collaboration among academic, government, and industry researchers focused on producing genomic tools for weedy species from around the world. Through this collaboration, our initial aim is to provide chromosome-level reference genome assemblies for at least 50 important weedy species from across the globe that are chosen based on member input, economic impact, and global prevalence (Fig. 1 ). Each genome will include annotation of gene models and repetitive elements and will be freely available through public databases with no intellectual property restrictions. Additionally, future funding of the IWGC will focus on improving gene annotations and supplementing these reference genomes with tools that increase their utility.
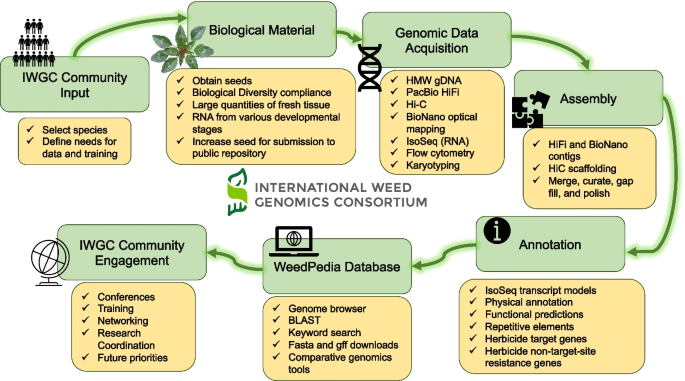
The International Weed Genomics Consortium (IWGC) collected input from the weed genomics community to develop plans for weed genome sequencing, annotation, user-friendly genome analysis tools, and community engagement
Reference genomes and data analysis tools
The first objective of the IWGC is to provide high-quality genomic resources for agriculturally important weeds. The IWGC therefore created two main resources for information about, access to, or analysis of weed genomic data (Fig. 1 ). The IWGC website (available at [ 167 ]) communicates the status and results of genome sequencing projects, information on training and funding opportunities, upcoming events, and news in weed genomics. It also contains details of all sequenced species including genome size, ploidy, chromosome number, herbicide resistance status, and reference genome assembly statistics. The IWGC either compiles existing data on genome size, ploidy, and chromosome number, or obtains the data using flow cytometry and cytogenetics (Fig. 1 ; Additional File 2 : Fig S1-S4). Through this website, users can request an account to access our second main resource, an online genome database called WeedPedia (accessible at [ 168 ]), with an account that is created within 3–5 working days of an account request submission. WeedPedia hosts IWGC-generated and other relevant publicly accessible genomic data as well as a suite of bioinformatic tools. Unlike what is available for other fields, weed science did not have a centralized hub for genomics information, data, and analysis prior to the IWGC. Our intention in creating WeedPedia is to encourage collaboration and equity of access to information across the research community. Importantly, all genome assemblies and annotations from the IWGC (Table 1 ), along with the raw data used to produce them, will be made available through NCBI GenBank. Upon completion of a 1-year sponsoring member data confidentiality period for each species (dates listed in Table 1 ), scientific teams within the IWGC produce the first genome-wide investigation to submit for publication including whole genome level analyses on genes, gene families, and repetitive sequences as well as comparative analysis with other species. Genome assemblies and data will be publicly available through NCBI as part of these initial publications for each species.
WeedPedia is a cloud-based omics database management platform built from the software “CropPedia” and licensed from KeyGene (Wageningen, The Netherlands). The interface allows users to access, visualize, and download genome assemblies along with structural and functional annotation. The platform includes a genome browser, comparative map viewer, pangenome tools, RNA-sequencing data visualization tools, genetic mapping and marker analysis tools, and alignment capabilities that allow searches by keyword or sequence. Additionally, genes encoding known target sites of herbicides have been specially annotated, allowing users to quickly identify and compare these genes of interest. The platform is flexible, making it compatible with future integration of other data types such as epigenetic or proteomic information. As an online platform with a graphical user interface, WeedPedia provides user-friendly, intuitive tools that encourage users to integrate genomics into their research while also allowing more advanced users to download genomic data to be used in custom analysis pipelines. We aspire for WeedPedia to mimic the success of other public genomic databases such as NCBI, CoGe, Phytozome, InsectBase, and Mycocosm to name a few. WeedPedia currently hosts reference genomes for 40 species (some of which are currently in their 1-year confidentiality period) with additional genomes in the pipeline to reach a currently planned total of 55 species (Table 1 ). These genomes include both de novo reference genomes generated or in progress by the IWGC (31 species; Table 1 ), and publicly available genome assemblies of 24 weedy or related species that were generated by independent research groups (Table 2 ). As of May 2024, WeedPedia has over 370 registered users from more than 27 countries spread across 6 continents.
The IWGC reference genomes are generated in partnership with the Corteva Agriscience Genome Center of Excellence (Johnston, Iowa) using a combination of single-molecule long-read sequencing, optical genome maps, and chromosome conformation mapping. This strategy has already yielded highly contiguous, phased, chromosome-level assemblies for 26 weed species, with additional assemblies currently in progress (Table 1 ). The IWGC assemblies have been completed as single or haplotype-resolved double-haplotype pseudomolecules in inbreeding and outbreeding species, respectively, with multiple genomes being near gapless. For example, the de novo assemblies of the allohexaploids Conyza sumatrensis and Chenopodium album have all chromosomes captured in single scaffolds and most chromosomes being gapless from telomere to telomere. Complementary full-length isoform (IsoSeq) sequencing of RNA collected from diverse tissue types and developmental stages assists in the development of gene models during annotation.
As with accessibility of data, a core objective of the IWGC is to facilitate open access to sequenced germplasm when possible for featured species. Historically, the weed science community has rarely shared or adopted standard germplasm (e.g., specific weed accessions). The IWGC has selected a specific accession of each species for reference genome assembly (typically susceptible to herbicides). In collaboration with a parallel effort by the Herbicide Resistant Plants committee of the Weed Science Society of America, seeds of the sequenced weed accessions will be deposited in the United States Department of Agriculture Germplasm Resources Information Network [ 186 ] for broad access by the scientific community and their accession numbers will be listed on the IWGC website. In some cases, it is not possible to generate enough seed to deposit into a public repository (e.g., plants that typically reproduce vegetatively, that are self-incompatible, or that produce very few seeds from a single individual). In these cases, the location of collection for sequenced accessions will at least inform the community where the sequenced individual came from and where they may expect to collect individuals with similar genotypes. The IWGC ensures that sequenced accessions are collected and documented to comply with the Nagoya Protocol on access to genetic resources and the fair and equitable sharing of benefits arising from their utilization under the Convention on Biological Diversity and related Access and Benefit Sharing Legislation [ 187 ]. As additional accessions of weed species are sequenced (e.g., pangenomes are obtained), the IWGC will facilitate germplasm sharing protocols to support collaboration. Further, to simplify the investigation of herbicide resistance, the IWGC will link WeedPedia with the International Herbicide-Resistant Weed Database [ 104 ], an already widely known and utilized database for weed scientists.
Training and collaboration in weed genomics
Beyond producing genomic tools and resources, a priority of the IWGC is to enable the utilization of these resources across a wide range of stakeholders. A holistic approach to training is required for weed science generally [ 188 ], and we would argue even more so for weed genomics. To accomplish our training goals, the IWGC is developing and delivering programs aimed at the full range of IWGC stakeholders and covering a breadth of relevant topics. We have taken care to ensure our approaches are diverse as to provide training to researchers with all levels of existing experience and differing reasons for engaging with these tools. Throughout, the focus is on ensuring that our training and outreach result in impacts that benefit a wide range of stakeholders.
Although recently developed tools are incredibly enabling and have great potential to replace antiquated methodology [ 189 ] and to solve pressing weed science problems [ 14 ], specialized computational skills are required to fully explore and unlock meaning from these highly complex datasets. Collaboration with, or training of, computational biologists equipped with these skills and resources developed by the IWGC will enable weed scientists to expand research programs and better understand the genetic underpinnings of weed evolution and herbicide resistance. To fill existing skill gaps, the IWGC is developing summer bootcamps and online modules directed specifically at weed scientists that will provide training on computational skills (Fig. 1 ). Because successful utilization of the IWGC resources requires more than general computational skills, we have created three targeted workshops that teach practical skills related to genomics databases, molecular biology, and population genomics (available at [ 190 ]). The IWGC has also hosted two official conference meetings, one in September of 2021 and one in January of 2023, with more conferences planned. These conferences have included invited speakers to present successful implementations of weed genomics, educational workshops to build computational skills, and networking opportunities for research to connect and collaborate.
Engagement opportunities during undergraduate degrees have been shown to improve academic outcomes [ 191 , 192 ]. As one activity to help achieve this goal, the IWGC has sponsored opportunities for US undergraduates to undertake a 10-week research experience, which includes an introduction to bioinformatics, a plant genomics research project that results in a presentation, and access to career building opportunities in diverse workplace environments. To increase equitable access to conferences and professional communities, we supported early career researchers to attend the first two IWGC conferences in the USA as well as workshops and bootcamps in Europe, South America, and Australia. These hybrid or in-person travel grants are intentionally designed to remove barriers and increase participation of individuals from backgrounds and experiences currently underrepresented within weed/plant science or genomics [ 193 ]. Recipients of these travel awards gave presentations and gained the measurable benefits that come from either virtual or in-person participation in conferences [ 194 ]. Moving forward, weed scientists must amass skills associated with genomic analyses and collaborate with other area experts to fully leverage resources developed by the IWGC.
The tools generated through the IWGC will enable many new research projects with diverse objectives like those listed above. In summary, contiguous genome assemblies and complete annotation information will allow weed scientists to join plant breeders in the use of genetic mapping for many traits including stress tolerance, plant architecture, and herbicide resistance (especially important for cases of NTSR). These assemblies will also allow for investigations of population structure, gene flow, and responses to evolutionary mechanisms like genetic bottlenecking and artificial selection. Understanding gene sequences across diverse weed species will be vital in modeling new herbicide target site proteins and designing novel effective herbicides with minimal off-target effects. The IWGC website will improve accessibility to weed genomics data by providing a single hub for reference genomes as well as phenotypic and genotypic information for accessions shared with the IWGC. Deposition of sequenced germplasm into public repositories will ensure that researchers are able to access and utilize these accessions in their own research to make the field more standardized and equitable. WeedPedia allows users of all backgrounds to quickly access information of interest such as herbicide target site gene sequence or subcellular localization of protein products for different genes. Users can also utilize server-based tools such as BLAST and genome browsing similar to other public genomic databases. Finally, the IWGC is committed to training and connecting weed genomicists through hosting trainings, workshops, and conferences.
Conclusions
Weeds are unique and fascinating plants, having significant impacts on agriculture and ecosystems; and yet, aspects of their biology, ecology, and genetics remain poorly understood. Weeds represent a unique area within plant biology, given their repeated rapid adaptation to sudden and severe shifts in the selective landscape of anthropogenic management practices. The production of a public genomics database with reference genomes and annotations for over 50 weed species represents a substantial step forward towards research goals that improve our understanding of the biology and evolution of weeds. Future work is needed to improve annotations, particularly for complex gene families involved in herbicide detoxification, structural variants, and mobile genetic elements. As reference genome assemblies become available; standard, affordable methods for gathering genotype information will allow for the identification of genetic variants underlying traits of interest. Further, methods for functional validation and hypothesis testing are needed in weeds to validate the effect of genetic variants detected through such experiments, including systems for transformation, gene editing, and transient gene silencing and expression. Future research should focus on utilizing weed genomes to investigate questions about evolutionary biology, ecology, genetics of weedy traits, and weed population dynamics. The IWGC plans to continue the public–private partnership model to host the WeedPedia database over time, integrate new datasets such as genome resequencing and transcriptomes, conduct trainings, and serve as a research coordination network to ensure that advances in weed science from around the world are shared across the research community (Fig. 1 ). Bridging basic plant genomics with translational applications in weeds is needed to deliver on the potential of weed genomics to improve weed management and crop breeding.
Availability of data and materials
All genome assemblies and related sequencing data produced by the IWGC will be available through NCBI as part of publications reporting the first genome-wide analysis for each species.
Gianessi LP, Nathan PR. The value of herbicides in U.S. crop production. Weed Technol. 2007;21(2):559–66.
Article Google Scholar
Pimentel D, Lach L, Zuniga R, Morrison D. Environmental and economic costs of nonindigenous species in the United States. Bioscience. 2000;50(1):53–65.
Barrett SH. Crop mimicry in weeds. Econ Bot. 1983;37(3):255–82.
Powles SB, Yu Q. Evolution in action: plants resistant to herbicides. Annu Rev Plant Biol. 2010;61:317–47.
Article CAS PubMed Google Scholar
Thurber CS, Reagon M, Gross BL, Olsen KM, Jia Y, Caicedo AL. Molecular evolution of shattering loci in U.S. weedy rice. Mol Ecol. 2010;19(16):3271–84.
Article PubMed PubMed Central Google Scholar
Comont D, Lowe C, Hull R, Crook L, Hicks HL, Onkokesung N, et al. Evolution of generalist resistance to herbicide mixtures reveals a trade-off in resistance management. Nat Commun. 2020;11(1):3086.
Article CAS PubMed PubMed Central Google Scholar
Ashworth MB, Walsh MJ, Flower KC, Vila-Aiub MM, Powles SB. Directional selection for flowering time leads to adaptive evolution in Raphanus raphanistrum (wild radish). Evol Appl. 2016;9(4):619–29.
Chan EK, Rowe HC, Kliebenstein DJ. Understanding the evolution of defense metabolites in Arabidopsis thaliana using genome-wide association mapping. Genetics. 2010;185(3):991–1007.
Frayling TM, Timpson NJ, Weedon MN, Zeggini E, Freathy RM, Lindgren CM, et al. A common variant in the FTO gene is associated with body mass index and predisposes to childhood and adult obesity. Science. 2007;316(5826):889–94.
Harkess A, Zhou J, Xu C, Bowers JE, Van der Hulst R, Ayyampalayam S, et al. The asparagus genome sheds light on the origin and evolution of a young Y chromosome. Nat Commun. 2017;8(1):1279.
Periyannan S, Moore J, Ayliffe M, Bansal U, Wang X, Huang L, et al. The gene Sr33 , an ortholog of barley Mla genes, encodes resistance to wheat stem rust race Ug99. Science. 2013;341(6147):786–8.
Ågren J, Oakley CG, McKay JK, Lovell JT, Schemske DW. Genetic mapping of adaptation reveals fitness tradeoffs in Arabidopsis thaliana . Proc Natl Acad Sci U S A. 2013;110(52):21077–82.
Article PubMed Central Google Scholar
Schartl M, Walter RB, Shen Y, Garcia T, Catchen J, Amores A, et al. The genome of the platyfish, Xiphophorus maculatus , provides insights into evolutionary adaptation and several complex traits. Nat Genet. 2013;45(5):567–72.
Ravet K, Patterson EL, Krähmer H, Hamouzová K, Fan L, Jasieniuk M, et al. The power and potential of genomics in weed biology and management. Pest Manag Sci. 2018;74(10):2216–25.
Hufford MB, Seetharam AS, Woodhouse MR, Chougule KM, Ou S, Liu J, et al. De novo assembly, annotation, and comparative analysis of 26 diverse maize genomes. Science. 2021;373(6555):655–62.
Liao W-W, Asri M, Ebler J, Doerr D, Haukness M, Hickey G, et al. A draft human pangenome reference. Nature. 2023;617(7960):312–24.
Huang Y, Wu D, Huang Z, Li X, Merotto A, Bai L, et al. Weed genomics: yielding insights into the genetics of weedy traits for crop improvement. aBIOTECH. 2023;4:20–30.
Chen K, Yang H, Wu D, Peng Y, Lian L, Bai L, et al. Weed biology and management in the multi-omics era: progress and perspectives. Plant Commun. 2024;5(4):100816.
De Wet JMJ, Harlan JR. Weeds and domesticates: evolution in the man-made habitat. Econ Bot. 1975;29(2):99–108.
Mahaut L, Cheptou PO, Fried G, Munoz F, Storkey J, Vasseur F, et al. Weeds: against the rules? Trends Plant Sci. 2020;25(11):1107–16.
Neve P, Vila-Aiub M, Roux F. Evolutionary-thinking in agricultural weed management. New Phytol. 2009;184(4):783–93.
Article PubMed Google Scholar
Sharma G, Barney JN, Westwood JH, Haak DC. Into the weeds: new insights in plant stress. Trends Plant Sci. 2021;26(10):1050–60.
Vigueira CC, Olsen KM, Caicedo AL. The red queen in the corn: agricultural weeds as models of rapid adaptive evolution. Heredity (Edinb). 2013;110(4):303–11.
Donohue K, Dorn L, Griffith C, Kim E, Aguilera A, Polisetty CR, et al. Niche construction through germination cueing: life-history responses to timing of germination in Arabidopsis thaliana . Evolution. 2005;59(4):771–85.
PubMed Google Scholar
Exposito-Alonso M. Seasonal timing adaptation across the geographic range of Arabidopsis thaliana . Proc Natl Acad Sci U S A. 2020;117(18):9665–7.
Fournier-Level A, Korte A, Cooper MD, Nordborg M, Schmitt J, Wilczek AM. A map of local adaptation in Arabidopsis thaliana . Science. 2011;334(6052):86–9.
Hancock AM, Brachi B, Faure N, Horton MW, Jarymowycz LB, Sperone FG, et al. Adaptation to climate across the Arabidopsis thaliana genome. Science. 2011;334(6052):83–6.
Initiative TAG. Analysis of the genome sequence of the flowering plant Arabidopsis thaliana . Nature. 2000;408(6814):796–815.
Alonso-Blanco C, Andrade J, Becker C, Bemm F, Bergelson J, Borgwardt KM, et al. 1,135 genomes reveal the global pattern of polymorphism in Arabidopsis thaliana . Cell. 2016;166(2):481–91.
Durvasula A, Fulgione A, Gutaker RM, Alacakaptan SI, Flood PJ, Neto C, et al. African genomes illuminate the early history and transition to selfing in Arabidopsis thaliana . Proc Natl Acad Sci U S A. 2017;114(20):5213–8.
Frachon L, Mayjonade B, Bartoli C, Hautekèete N-C, Roux F. Adaptation to plant communities across the genome of Arabidopsis thaliana . Mol Biol Evol. 2019;36(7):1442–56.
Fulgione A, Koornneef M, Roux F, Hermisson J, Hancock AM. Madeiran Arabidopsis thaliana reveals ancient long-range colonization and clarifies demography in Eurasia. Mol Biol Evol. 2018;35(3):564–74.
Fulgione A, Neto C, Elfarargi AF, Tergemina E, Ansari S, Göktay M, et al. Parallel reduction in flowering time from de novo mutations enable evolutionary rescue in colonizing lineages. Nat Commun. 2022;13(1):1461.
Kasulin L, Rowan BA, León RJC, Schuenemann VJ, Weigel D, Botto JF. A single haplotype hyposensitive to light and requiring strong vernalization dominates Arabidopsis thaliana populations in Patagonia. Argentina Mol Ecol. 2017;26(13):3389–404.
Picó FX, Méndez-Vigo B, Martínez-Zapater JM, Alonso-Blanco C. Natural genetic variation of Arabidopsis thaliana is geographically structured in the Iberian peninsula. Genetics. 2008;180(2):1009–21.
Atwell S, Huang YS, Vilhjálmsson BJ, Willems G, Horton M, Li Y, et al. Genome-wide association study of 107 phenotypes in Arabidopsis thaliana inbred lines. Nature. 2010;465(7298):627–31.
Flood PJ, Kruijer W, Schnabel SK, van der Schoor R, Jalink H, Snel JFH, et al. Phenomics for photosynthesis, growth and reflectance in Arabidopsis thaliana reveals circadian and long-term fluctuations in heritability. Plant Methods. 2016;12(1):14.
Marchadier E, Hanemian M, Tisné S, Bach L, Bazakos C, Gilbault E, et al. The complex genetic architecture of shoot growth natural variation in Arabidopsis thaliana . PLoS Genet. 2019;15(4):e1007954.
Tisné S, Serrand Y, Bach L, Gilbault E, Ben Ameur R, Balasse H, et al. Phenoscope: an automated large-scale phenotyping platform offering high spatial homogeneity. Plant J. 2013;74(3):534–44.
Tschiersch H, Junker A, Meyer RC, Altmann T. Establishment of integrated protocols for automated high throughput kinetic chlorophyll fluorescence analyses. Plant Methods. 2017;13:54.
Chen X, MacGregor DR, Stefanato FL, Zhang N, Barros-Galvão T, Penfield S. A VEL3 histone deacetylase complex establishes a maternal epigenetic state controlling progeny seed dormancy. Nat Commun. 2023;14(1):2220.
Choi M, Scholl UI, Ji W, Liu T, Tikhonova IR, Zumbo P, et al. Genetic diagnosis by whole exome capture and massively parallel DNA sequencing. Proc Natl Acad Sci U S A. 2009;106(45):19096–101.
Davey JW, Blaxter ML. RADSeq: next-generation population genetics. Brief Funct Genomics. 2010;9(5–6):416–23.
Elshire RJ, Glaubitz JC, Sun Q, Poland JA, Kawamoto K, Buckler ES, et al. A robust, simple genotyping-by-sequencing (GBS) approach for high diversity species. PLoS ONE. 2011;6(5):e19379.
MacGregor DR. What makes a weed a weed? How virus-mediated reverse genetics can help to explore the genetics of weediness. Outlooks Pest Manag. 2020;31(5):224–9.
Mellado-Sánchez M, McDiarmid F, Cardoso V, Kanyuka K, MacGregor DR. Virus-mediated transient expression techniques enable gene function studies in blackgrass. Plant Physiol. 2020;183(2):455–9.
Dimaano NG, Yamaguchi T, Fukunishi K, Tominaga T, Iwakami S. Functional characterization of Cytochrome P450 CYP81A subfamily to disclose the pattern of cross-resistance in Echinochloa phyllopogon . Plant Mol Biol. 2020;102(4–5):403–16.
de Figueiredo MRA, Küpper A, Malone JM, Petrovic T, de Figueiredo ABTB, Campagnola G, et al. An in-frame deletion mutation in the degron tail of auxin coreceptor IAA2 confers resistance to the herbicide 2,4-D in Sisymbrium orientale . Proc Natl Acad Sci U S A. 2022;119(9):e2105819119.
Patzoldt WL, Hager AG, McCormick JS, Tranel PJ. A codon deletion confers resistance to herbicides inhibiting protoporphyrinogen oxidase. Proc Natl Acad Sci U S A. 2006;103(33):12329–34.
Zabala-Pardo D, Gaines T, Lamego FP, Avila LA. RNAi as a tool for weed management: challenges and opportunities. Adv Weed Sci. 2022;40(spe1):e020220096.
Fattorini R, Glover BJ. Molecular mechanisms of pollination biology. Annu Rev Plant Biol. 2020;71:487–515.
Rollin O, Benelli G, Benvenuti S, Decourtye A, Wratten SD, Canale A, et al. Weed-insect pollinator networks as bio-indicators of ecological sustainability in agriculture. A review Agron Sustain Dev. 2016;36(1):8.
Irwin RE, Strauss SY. Flower color microevolution in wild radish: evolutionary response to pollinator-mediated selection. Am Nat. 2005;165(2):225–37.
Ma B, Wu J, Shi T-L, Yang Y-Y, Wang W-B, Zheng Y, et al. Lilac ( Syringa oblata ) genome provides insights into its evolution and molecular mechanism of petal color change. Commun Biol. 2022;5(1):686.
Xing A, Wang X, Nazir MF, Zhang X, Wang X, Yang R, et al. Transcriptomic and metabolomic profiling of flavonoid biosynthesis provides novel insights into petals coloration in Asian cotton ( Gossypium arboreum L.). BMC Plant Biol. 2022;22(1):416.
Zheng Y, Chen Y, Liu Z, Wu H, Jiao F, Xin H, et al. Important roles of key genes and transcription factors in flower color differences of Nicotiana alata . Genes (Basel). 2021;12(12):1976.
Krizek BA, Anderson JT. Control of flower size. J Exp Bot. 2013;64(6):1427–37.
Powell AE, Lenhard M. Control of organ size in plants. Curr Biol. 2012;22(9):R360–7.
Spencer V, Kim M. Re"CYC"ling molecular regulators in the evolution and development of flower symmetry. Semin Cell Dev Biol. 2018;79:16–26.
Amrad A, Moser M, Mandel T, de Vries M, Schuurink RC, Freitas L, et al. Gain and loss of floral scent production through changes in structural genes during pollinator-mediated speciation. Curr Biol. 2016;26(24):3303–12.
Delle-Vedove R, Schatz B, Dufay M. Understanding intraspecific variation of floral scent in light of evolutionary ecology. Ann Bot. 2017;120(1):1–20.
Pichersky E, Gershenzon J. The formation and function of plant volatiles: perfumes for pollinator attraction and defense. Curr Opin Plant Biol. 2002;5(3):237–43.
Ballerini ES, Kramer EM, Hodges SA. Comparative transcriptomics of early petal development across four diverse species of Aquilegia reveal few genes consistently associated with nectar spur development. BMC Genom. 2019;20(1):668.
Corbet SA, Willmer PG, Beament JWL, Unwin DM, Prys-Jones OE. Post-secretory determinants of sugar concentration in nectar. Plant Cell Environ. 1979;2(4):293–308.
Galliot C, Hoballah ME, Kuhlemeier C, Stuurman J. Genetics of flower size and nectar volume in Petunia pollination syndromes. Planta. 2006;225(1):203–12.
Vila-Aiub MM, Neve P, Powles SB. Fitness costs associated with evolved herbicide resistance alleles in plants. New Phytol. 2009;184(4):751–67.
Baucom RS. Evolutionary and ecological insights from herbicide-resistant weeds: what have we learned about plant adaptation, and what is left to uncover? New Phytol. 2019;223(1):68–82.
Bajwa AA, Latif S, Borger C, Iqbal N, Asaduzzaman M, Wu H, et al. The remarkable journey of a weed: biology and management of annual ryegrass ( Lolium rigidum ) in conservation cropping systems of Australia. Plants (Basel). 2021;10(8):1505.
Bitarafan Z, Andreasen C. Fecundity allocation in some european weed species competing with crops. Agronomy. 2022;12(5):1196.
Costea M, Weaver SE, Tardif FJ. The biology of Canadian weeds. 130. Amaranthus retroflexus L., A. powellii , A. powellii S. Watson, and A. hybridus L. Can J Plant Sci. 2004;84(2):631–68.
Dixon A, Comont D, Slavov GT, Neve P. Population genomics of selectively neutral genetic structure and herbicide resistance in UK populations of Alopecurus myosuroides . Pest Manag Sci. 2021;77(3):1520–9.
Kersten S, Chang J, Huber CD, Voichek Y, Lanz C, Hagmaier T, et al. Standing genetic variation fuels rapid evolution of herbicide resistance in blackgrass. Proc Natl Acad Sci U S A. 2023;120(16):e2206808120.
Qiu J, Zhou Y, Mao L, Ye C, Wang W, Zhang J, et al. Genomic variation associated with local adaptation of weedy rice during de-domestication. Nat Commun. 2017;8(1):15323.
Kreiner JM, Caballero A, Wright SI, Stinchcombe JR. Selective ancestral sorting and de novo evolution in the agricultural invasion of Amaranthus tuberculatus . Evolution. 2022;76(1):70–85.
Kreiner JM, Latorre SM, Burbano HA, Stinchcombe JR, Otto SP, Weigel D, et al. Rapid weed adaptation and range expansion in response to agriculture over the past two centuries. Science. 2022;378(6624):1079–85.
Wu D, Shen E, Jiang B, Feng Y, Tang W, Lao S, et al. Genomic insights into the evolution of Echinochloa species as weed and orphan crop. Nat Commun. 2022;13(1):689.
Yeaman S, Hodgins KA, Lotterhos KE, Suren H, Nadeau S, Degner JC, et al. Convergent local adaptation to climate in distantly related conifers. Science. 2016;353(6306):1431–3.
Haudry A, Platts AE, Vello E, Hoen DR, Leclercq M, Williamson RJ, et al. An atlas of over 90,000 conserved noncoding sequences provides insight into crucifer regulatory regions. Nat Genet. 2013;45(8):891–8.
Sackton TB, Grayson P, Cloutier A, Hu Z, Liu JS, Wheeler NE, et al. Convergent regulatory evolution and loss of flight in paleognathous birds. Science. 2019;364(6435):74–8.
Ye CY, Fan L. Orphan crops and their wild relatives in the genomic era. Mol Plant. 2021;14(1):27–39.
Clements DR, Jones VL. Ten ways that weed evolution defies human management efforts amidst a changing climate. Agronomy. 2021;11(2):284.
Article CAS Google Scholar
Weinig C. Rapid evolutionary responses to selection in heterogeneous environments among agricultural and nonagricultural weeds. Int J Plant Sci. 2005;166(4):641–7.
Cousens RD, Fournier-Level A. Herbicide resistance costs: what are we actually measuring and why? Pest Manag Sci. 2018;74(7):1539–46.
Lasky JR, Josephs EB, Morris GP. Genotype–environment associations to reveal the molecular basis of environmental adaptation. Plant Cell. 2023;35(1):125–38.
Lotterhos KE. The effect of neutral recombination variation on genome scans for selection. G3-Genes Genom Genet. 2019;9(6):1851–67.
Lovell JT, MacQueen AH, Mamidi S, Bonnette J, Jenkins J, Napier JD, et al. Genomic mechanisms of climate adaptation in polyploid bioenergy switchgrass. Nature. 2021;590(7846):438–44.
Todesco M, Owens GL, Bercovich N, Légaré J-S, Soudi S, Burge DO, et al. Massive haplotypes underlie ecotypic differentiation in sunflowers. Nature. 2020;584(7822):602–7.
Revolinski SR, Maughan PJ, Coleman CE, Burke IC. Preadapted to adapt: Underpinnings of adaptive plasticity revealed by the downy brome genome. Commun Biol. 2023;6(1):326.
Kuester A, Conner JK, Culley T, Baucom RS. How weeds emerge: a taxonomic and trait-based examination using United States data. New Phytol. 2014;202(3):1055–68.
Arnaud JF, Fénart S, Cordellier M, Cuguen J. Populations of weedy crop-wild hybrid beets show contrasting variation in mating system and population genetic structure. Evol Appl. 2010;3(3):305–18.
Ellstrand NC, Schierenbeck KA. Hybridization as a stimulus for the evolution of invasiveness in plants? Proc Natl Acad Sci U S A. 2000;97(13):7043–50.
Nakabayashi K, Leubner-Metzger G. Seed dormancy and weed emergence: from simulating environmental change to understanding trait plasticity, adaptive evolution, and population fitness. J Exp Bot. 2021;72(12):4181–5.
Busi R, Yu Q, Barrett-Lennard R, Powles S. Long distance pollen-mediated flow of herbicide resistance genes in Lolium rigidum . Theor Appl Genet. 2008;117(8):1281–90.
Délye C, Clément JAJ, Pernin F, Chauvel B, Le Corre V. High gene flow promotes the genetic homogeneity of arable weed populations at the landscape level. Basic Appl Ecol. 2010;11(6):504–12.
Roumet M, Noilhan C, Latreille M, David J, Muller MH. How to escape from crop-to-weed gene flow: phenological variation and isolation-by-time within weedy sunflower populations. New Phytol. 2013;197(2):642–54.
Moghadam SH, Alebrahim MT, Mohebodini M, MacGregor DR. Genetic variation of Amaranthus retroflexus L. and Chenopodium album L. (Amaranthaceae) suggests multiple independent introductions into Iran. Front Plant Sci. 2023;13:1024555.
Muller M-H, Latreille M, Tollon C. The origin and evolution of a recent agricultural weed: population genetic diversity of weedy populations of sunflower ( Helianthus annuus L.) in Spain and France. Evol Appl. 2011;4(3):499–514.
Wesse C, Welk E, Hurka H, Neuffer B. Geographical pattern of genetic diversity in Capsella bursa-pastoris (Brassicaceae) -A global perspective. Ecol Evol. 2021;11(1):199–213.
Fraimout A, Debat V, Fellous S, Hufbauer RA, Foucaud J, Pudlo P, et al. Deciphering the routes of invasion of Drosophila suzukii by means of ABC random forest. Mol Biol Evol. 2017;34(4):980–96.
CAS PubMed PubMed Central Google Scholar
Battlay P, Wilson J, Bieker VC, Lee C, Prapas D, Petersen B, et al. Large haploblocks underlie rapid adaptation in the invasive weed Ambrosia artemisiifolia . Nat Commun. 2023;14(1):1717.
van Boheemen LA, Hodgins KA. Rapid repeatable phenotypic and genomic adaptation following multiple introductions. Mol Ecol. 2020;29(21):4102–17.
Putra A, Hodgins K, Fournier-Level A. Assessing the invasive potential of different source populations of ragweed ( Ambrosia artemisiifolia L.) through genomically-informed species distribution modelling. Authorea. 2023;17(1):e13632.
Google Scholar
Bourguet D, Delmotte F, Franck P, Guillemaud T, Reboud X, Vacher C, et al. Heterogeneity of selection and the evolution of resistance. Trends Ecol Evol. 2013;28(2):110–8.
The International Herbicide-Resistant Weed Database. www.weedscience.org . Accessed 20 June 2023.
Powles S. Herbicide discovery through innovation and diversity. Adv Weed Sci. 2022;40(spe1):e020220074.
Murphy BP, Tranel PJ. Target-site mutations conferring herbicide resistance. Plants (Basel). 2019;8(10):382.
Gaines TA, Duke SO, Morran S, Rigon CAG, Tranel PJ, Küpper A, et al. Mechanisms of evolved herbicide resistance. J Biol Chem. 2020;295(30):10307–30.
Lonhienne T, Cheng Y, Garcia MD, Hu SH, Low YS, Schenk G, et al. Structural basis of resistance to herbicides that target acetohydroxyacid synthase. Nat Commun. 2022;13(1):3368.
Comont D, MacGregor DR, Crook L, Hull R, Nguyen L, Freckleton RP, et al. Dissecting weed adaptation: fitness and trait correlations in herbicide-resistant Alopecurus myosuroides . Pest Manag Sci. 2022;78(7):3039–50.
Neve P. Simulation modelling to understand the evolution and management of glyphosate resistance in weeds. Pest Manag Sci. 2008;64(4):392–401.
Torra J, Alcántara-de la Cruz R. Molecular mechanisms of herbicide resistance in weeds. Genes (Basel). 2022;13(11):2025.
Délye C, Gardin JAC, Boucansaud K, Chauvel B, Petit C. Non-target-site-based resistance should be the centre of attention for herbicide resistance research: Alopecurus myosuroides as an illustration. Weed Res. 2011;51(5):433–7.
Chandra S, Leon RG. Genome-wide evolutionary analysis of putative non-specific herbicide resistance genes and compilation of core promoters between monocots and dicots. Genes (Basel). 2022;13(7):1171.
Margaritopoulou T, Tani E, Chachalis D, Travlos I. Involvement of epigenetic mechanisms in herbicide resistance: the case of Conyza canadensis . Agriculture. 2018;8(1):17.
Pan L, Guo Q, Wang J, Shi L, Yang X, Zhou Y, et al. CYP81A68 confers metabolic resistance to ALS and ACCase-inhibiting herbicides and its epigenetic regulation in Echinochloa crus-galli . J Hazard Mater. 2022;428:128225.
Sen MK, Hamouzová K, Košnarová P, Roy A, Soukup J. Herbicide resistance in grass weeds: Epigenetic regulation matters too. Front Plant Sci. 2022;13:1040958.
Han H, Yu Q, Beffa R, González S, Maiwald F, Wang J, et al. Cytochrome P450 CYP81A10v7 in Lolium rigidum confers metabolic resistance to herbicides across at least five modes of action. Plant J. 2021;105(1):79–92.
Kubis GC, Marques RZ, Kitamura RS, Barroso AA, Juneau P, Gomes MP. Antioxidant enzyme and Cytochrome P450 activities are involved in horseweed ( Conyza sumatrensis ) resistance to glyphosate. Stress. 2023;3(1):47–57.
Qiao Y, Zhang N, Liu J, Yang H. Interpretation of ametryn biodegradation in rice based on joint analyses of transcriptome, metabolome and chemo-characterization. J Hazard Mater. 2023;445:130526.
Rouse CE, Roma-Burgos N, Barbosa Martins BA. Physiological assessment of non–target site restistance in multiple-resistant junglerice ( Echinochloa colona ). Weed Sci. 2019;67(6):622–32.
Abou-Khater L, Maalouf F, Jighly A, Alsamman AM, Rubiales D, Rispail N, et al. Genomic regions associated with herbicide tolerance in a worldwide faba bean ( Vicia faba L.) collection. Sci Rep. 2022;12(1):158.
Gupta S, Harkess A, Soble A, Van Etten M, Leebens-Mack J, Baucom RS. Interchromosomal linkage disequilibrium and linked fitness cost loci associated with selection for herbicide resistance. New Phytol. 2023;238(3):1263–77.
Kreiner JM, Tranel PJ, Weigel D, Stinchcombe JR, Wright SI. The genetic architecture and population genomic signatures of glyphosate resistance in Amaranthus tuberculatus . Mol Ecol. 2021;30(21):5373–89.
Parcharidou E, Dücker R, Zöllner P, Ries S, Orru R, Beffa R. Recombinant glutathione transferases from flufenacet-resistant black-grass ( Alopecurus myosuroides Huds.) form different flufenacet metabolites and differ in their interaction with pre- and post-emergence herbicides. Pest Manag Sci. 2023;79(9):3376–86.
Békés M, Langley DR, Crews CM. PROTAC targeted protein degraders: the past is prologue. Nat Rev Drug Discov. 2022;21(3):181–200.
Acuner Ozbabacan SE, Engin HB, Gursoy A, Keskin O. Transient protein-protein interactions. Protein Eng Des Sel. 2011;24(9):635–48.
Lu H, Zhou Q, He J, Jiang Z, Peng C, Tong R, et al. Recent advances in the development of protein–protein interactions modulators: mechanisms and clinical trials. Signal Transduct Target Ther. 2020;5(1):213.
Benson CW, Sheltra MR, Maughan PJ, Jellen EN, Robbins MD, Bushman BS, et al. Homoeologous evolution of the allotetraploid genome of Poa annua L. BMC Genom. 2023;24(1):350.
Robbins MD, Bushman BS, Huff DR, Benson CW, Warnke SE, Maughan CA, et al. Chromosome-scale genome assembly and annotation of allotetraploid annual bluegrass ( Poa annua L.). Genome Biol Evol. 2022;15(1):evac180.
Montgomery JS, Giacomini D, Waithaka B, Lanz C, Murphy BP, Campe R, et al. Draft genomes of Amaranthus tuberculatus , Amaranthus hybridus and Amaranthus palmeri . Genome Biol Evol. 2020;12(11):1988–93.
Jeschke MR, Tranel PJ, Rayburn AL. DNA content analysis of smooth pigweed ( Amaranthus hybridus ) and tall waterhemp ( A. tuberculatus ): implications for hybrid detection. Weed Sci. 2003;51(1):1–3.
Rayburn AL, McCloskey R, Tatum TC, Bollero GA, Jeschke MR, Tranel PJ. Genome size analysis of weedy Amaranthus species. Crop Sci. 2005;45(6):2557–62.
Laforest M, Martin SL, Bisaillon K, Soufiane B, Meloche S, Tardif FJ, et al. The ancestral karyotype of the Heliantheae Alliance, herbicide resistance, and human allergens: Insights from the genomes of common and giant ragweed. Plant Genome . 2024;e20442. https://doi.org/10.1002/tpg2.20442 .
Mulligan GA. Chromosome numbers of Canadian weeds. I Canad J Bot. 1957;35(5):779–89.
Meyer L, Causse R, Pernin F, Scalone R, Bailly G, Chauvel B, et al. New gSSR and EST-SSR markers reveal high genetic diversity in the invasive plant Ambrosia artemisiifolia L. and can be transferred to other invasive Ambrosia species. PLoS One. 2017;12(5):e0176197.
Pustahija F, Brown SC, Bogunić F, Bašić N, Muratović E, Ollier S, et al. Small genomes dominate in plants growing on serpentine soils in West Balkans, an exhaustive study of 8 habitats covering 308 taxa. Plant Soil. 2013;373(1):427–53.
Kubešová M, Moravcova L, Suda J, Jarošík V, Pyšek P. Naturalized plants have smaller genomes than their non-invading relatives: a flow cytometric analysis of the Czech alien flora. Preslia. 2010;82(1):81–96.
Thébaud C, Abbott RJ. Characterization of invasive Conyza species (Asteraceae) in Europe: quantitative trait and isozyme analysis. Am J Bot. 1995;82(3):360–8.
Garcia S, Hidalgo O, Jakovljević I, Siljak-Yakovlev S, Vigo J, Garnatje T, et al. New data on genome size in 128 Asteraceae species and subspecies, with first assessments for 40 genera, 3 tribes and 2 subfamilies. Plant Biosyst. 2013;147(4):1219–27.
Zhao X, Yi L, Ren Y, Li J, Ren W, Hou Z, et al. Chromosome-scale genome assembly of the yellow nutsedge ( Cyperus esculentus ). Genome Biol Evol. 2023;15(3):evad027.
Bennett MD, Leitch IJ, Hanson L. DNA amounts in two samples of angiosperm weeds. Ann Bot. 1998;82:121–34.
Schulz-Schaeffer J, Gerhardt S. Cytotaxonomic analysis of the Euphorbia spp. (leafy spurge) complex. II: Comparative study of the chromosome morphology. Biol Zentralbl. 1989;108(1):69–76.
Schaeffer JR, Gerhardt S. The impact of introgressive hybridization on the weediness of leafy spurge. Leafy Spurge Symposium. 1989;1989:97–105.
Bai C, Alverson WS, Follansbee A, Waller DM. New reports of nuclear DNA content for 407 vascular plant taxa from the United States. Ann Bot. 2012;110(8):1623–9.
Aarestrup JR, Karam D, Fernandes GW. Chromosome number and cytogenetics of Euphorbia heterophylla L. Genet Mol Res. 2008;7(1):217–22.
Wang L, Sun X, Peng Y, Chen K, Wu S, Guo Y, et al. Genomic insights into the origin, adaptive evolution, and herbicide resistance of Leptochloa chinensis , a devastating tetraploid weedy grass in rice fields. Mol Plant. 2022;15(6):1045–58.
Paril J, Pandey G, Barnett EM, Rane RV, Court L, Walsh T, et al. Rounding up the annual ryegrass genome: high-quality reference genome of Lolium rigidum . Front Genet. 2022;13:1012694.
Weiss-Schneeweiss H, Greilhuber J, Schneeweiss GM. Genome size evolution in holoparasitic Orobanche (Orobanchaceae) and related genera. Am J Bot. 2006;93(1):148–56.
Towers G, Mitchell J, Rodriguez E, Bennett F, Subba Rao P. Biology & chemistry of Parthenium hysterophorus L., a problem weed in India. Biol Rev. 1977;48:65–74.
CAS Google Scholar
Moghe GD, Hufnagel DE, Tang H, Xiao Y, Dworkin I, Town CD, et al. Consequences of whole-genome triplication as revealed by comparative genomic analyses of the wild radish ( Raphanus raphanistrum ) and three other Brassicaceae species. Plant Cell. 2014;26(5):1925–37.
Zhang X, Liu T, Wang J, Wang P, Qiu Y, Zhao W, et al. Pan-genome of Raphanus highlights genetic variation and introgression among domesticated, wild, and weedy radishes. Mol Plant. 2021;14(12):2032–55.
Chytrý M, Danihelka J, Kaplan Z, Wild J, Holubová D, Novotný P, et al. Pladias database of the Czech flora and vegetation. Preslia. 2021;93(1):1–87.
Patterson EL, Pettinga DJ, Ravet K, Neve P, Gaines TA. Glyphosate resistance and EPSPS gene duplication: Convergent evolution in multiple plant species. J Hered. 2018;109(2):117–25.
Jugulam M, Niehues K, Godar AS, Koo DH, Danilova T, Friebe B, et al. Tandem amplification of a chromosomal segment harboring 5-enolpyruvylshikimate-3-phosphate synthase locus confers glyphosate resistance in Kochia scoparia . Plant Physiol. 2014;166(3):1200–7.
Patterson EL, Saski CA, Sloan DB, Tranel PJ, Westra P, Gaines TA. The draft genome of Kochia scoparia and the mechanism of glyphosate resistance via transposon-mediated EPSPS tandem gene duplication. Genome Biol Evol. 2019;11(10):2927–40.
Zhang C, Johnson N, Hall N, Tian X, Yu Q, Patterson E. Subtelomeric 5-enolpyruvylshikimate-3-phosphate synthase ( EPSPS ) copy number variation confers glyphosate resistance in Eleusine indica . Nat Commun. 2023;14:4865.
Koo D-H, Molin WT, Saski CA, Jiang J, Putta K, Jugulam M, et al. Extrachromosomal circular DNA-based amplification and transmission of herbicide resistance in crop weed Amaranthus palmeri . Proc Natl Acad Sci U S A. 2018;115(13):3332–7.
Molin WT, Yaguchi A, Blenner M, Saski CA. The eccDNA Replicon: A heritable, extranuclear vehicle that enables gene amplification and glyphosate resistance in Amaranthus palmeri . Plant Cell. 2020;32(7):2132–40.
Jugulam M. Can non-Mendelian inheritance of extrachromosomal circular DNA-mediated EPSPS gene amplification provide an opportunity to reverse resistance to glyphosate? Weed Res. 2021;61(2):100–5.
Kreiner JM, Giacomini DA, Bemm F, Waithaka B, Regalado J, Lanz C, et al. Multiple modes of convergent adaptation in the spread of glyphosate-resistant Amaranthus tuberculatus . Proc Natl Acad Sci U S A. 2019;116(42):21076–84.
Cai L, Comont D, MacGregor D, Lowe C, Beffa R, Neve P, et al. The blackgrass genome reveals patterns of non-parallel evolution of polygenic herbicide resistance. New Phytol. 2023;237(5):1891–907.
Chen K, Yang H, Peng Y, Liu D, Zhang J, Zhao Z, et al. Genomic analyses provide insights into the polyploidization-driven herbicide adaptation in Leptochloa weeds. Plant Biotechnol J. 2023;21(8):1642–58.
Ohadi S, Hodnett G, Rooney W, Bagavathiannan M. Gene flow and its consequences in Sorghum spp. Crit Rev Plant Sci. 2017;36(5–6):367–85.
Renzi JP, Coyne CJ, Berger J, von Wettberg E, Nelson M, Ureta S, et al. How could the use of crop wild relatives in breeding increase the adaptation of crops to marginal environments? Front Plant Sci. 2022;13:886162.
Ward SM, Cousens RD, Bagavathiannan MV, Barney JN, Beckie HJ, Busi R, et al. Agricultural weed research: a critique and two proposals. Weed Sci. 2014;62(4):672–8.
Evans JA, Tranel PJ, Hager AG, Schutte B, Wu C, Chatham LA, et al. Managing the evolution of herbicide resistance. Pest Manag Sci. 2016;72(1):74–80.
International Weed Genomics Consortium Website. https://www.weedgenomics.org . Accessed 20 June 2023.
WeedPedia Database. https://weedpedia.weedgenomics.org/ . Accessed 20 June 2023.
Hall N, Chen J, Matzrafi M, Saski CA, Westra P, Gaines TA, et al. FHY3/FAR1 transposable elements generate adaptive genetic variation in the Bassia scoparia genome. bioRxiv . 2023; DOI: https://doi.org/10.1101/2023.05.26.542497 .
Jarvis DE, Sproul JS, Navarro-Domínguez B, Krak K, Jaggi K, Huang Y-F, et al. Chromosome-scale genome assembly of the hexaploid Taiwanese goosefoot “Djulis” ( Chenopodium formosanum ). Genome Biol Evol. 2022;14(8):evac120.
Ferreira LAI, de Oliveira RS, Jr., Constantin J, Brunharo C. Evolution of ACCase-inhibitor resistance in Chloris virgata is conferred by a Trp2027Cys mutation in the herbicide target site. Pest Manag Sci. 2023;79(12):5220–9.
Laforest M, Martin SL, Bisaillon K, Soufiane B, Meloche S, Page E. A chromosome-scale draft sequence of the Canada fleabane genome. Pest Manag Sci. 2020;76(6):2158–69.
Guo L, Qiu J, Ye C, Jin G, Mao L, Zhang H, et al. Echinochloa crus-galli genome analysis provides insight into its adaptation and invasiveness as a weed. Nat Commun. 2017;8(1):1031.
Sato MP, Iwakami S, Fukunishi K, Sugiura K, Yasuda K, Isobe S, et al. Telomere-to-telomere genome assembly of an allotetraploid pernicious weed, Echinochloa phyllopogon . DNA Res. 2023;30(5):dsad023.
Stein JC, Yu Y, Copetti D, Zwickl DJ, Zhang L, Zhang C, et al. Genomes of 13 domesticated and wild rice relatives highlight genetic conservation, turnover and innovation across the genus Oryza . Nat Genet. 2018;50(2):285–96.
Wu D, Xie L, Sun Y, Huang Y, Jia L, Dong C, et al. A syntelog-based pan-genome provides insights into rice domestication and de-domestication. Genome Biol. 2023;24(1):179.
Wang Z, Huang S, Yang Z, Lai J, Gao X, Shi J. A high-quality, phased genome assembly of broomcorn millet reveals the features of its subgenome evolution and 3D chromatin organization. Plant Commun. 2023;4(3):100557.
Mao Q, Huff DR. The evolutionary origin of Poa annua L. Crop Sci. 2012;52(4):1910–22.
Benson CW, Sheltra MR, Maughan JP, Jellen EN, Robbins MD, Bushman BS, et al. Homoeologous evolution of the allotetraploid genome of Poa annua L. Res Sq. 2023. https://doi.org/10.21203/rs.3.rs-2729084/v1 .
Brunharo C, Benson CW, Huff DR, Lasky JR. Chromosome-scale genome assembly of Poa trivialis and population genomics reveal widespread gene flow in a cool-season grass seed production system. Plant Direct. 2024;8(3):e575.
Mo C, Wu Z, Shang X, Shi P, Wei M, Wang H, et al. Chromosome-level and graphic genomes provide insights into metabolism of bioactive metabolites and cold-adaption of Pueraria lobata var. montana . DNA Research. 2022;29(5):dsac030.
Thielen PM, Pendleton AL, Player RA, Bowden KV, Lawton TJ, Wisecaver JH. Reference genome for the highly transformable Setaria viridis ME034V. G3 (Bethesda, Md). 2020;10(10):3467–78.
Yoshida S, Kim S, Wafula EK, Tanskanen J, Kim Y-M, Honaas L, et al. Genome sequence of Striga asiatica provides insight into the evolution of plant parasitism. Curr Biol. 2019;29(18):3041–52.
Qiu S, Bradley JM, Zhang P, Chaudhuri R, Blaxter M, Butlin RK, et al. Genome-enabled discovery of candidate virulence loci in Striga hermonthica , a devastating parasite of African cereal crops. New Phytol. 2022;236(2):622–38.
Nunn A, Rodríguez-Arévalo I, Tandukar Z, Frels K, Contreras-Garrido A, Carbonell-Bejerano P, et al. Chromosome-level Thlaspi arvense genome provides new tools for translational research and for a newly domesticated cash cover crop of the cooler climates. Plant Biotechnol J. 2022;20(5):944–63.
USDA-ARS Germplasm Resources Information Network (GRIN). https://www.ars-grin.gov/ . Accessed 20 June 2023.
Buck M, Hamilton C. The Nagoya Protocol on access to genetic resources and the fair and equitable sharing of benefits arising from their utilization to the convention on biological diversity. RECIEL. 2011;20(1):47–61.
Chauhan BS, Matloob A, Mahajan G, Aslam F, Florentine SK, Jha P. Emerging challenges and opportunities for education and research in weed science. Front Plant Sci. 2017;8:1537.
Shah S, Lonhienne T, Murray CE, Chen Y, Dougan KE, Low YS, et al. Genome-guided analysis of seven weed species reveals conserved sequence and structural features of key gene targets for herbicide development. Front Plant Sci. 2022;13:909073.
International Weed Genomics Consortium Training Resources. https://www.weedgenomics.org/training-resources/ . Accessed 20 June 2023.
Blackford S. Harnessing the power of communities: career networking strategies for bioscience PhD students and postdoctoral researchers. FEMS Microbiol Lett. 2018;365(8):fny033.
Pender M, Marcotte DE, Sto Domingo MR, Maton KI. The STEM pipeline: The role of summer research experience in minority students’ Ph.D. aspirations. Educ Policy Anal Arch. 2010;18(30):1–36.
PubMed PubMed Central Google Scholar
Burke A, Okrent A, Hale K. The state of U.S. science and engineering 2022. Foundation NS. https://ncses.nsf.gov/pubs/nsb20221 . 2022.
Wu J-Y, Liao C-H, Cheng T, Nian M-W. Using data analytics to investigate attendees’ behaviors and psychological states in a virtual academic conference. Educ Technol Soc. 2021;24(1):75–91.
Download references
Peer review information
Wenjing She was the primary editor of this article and managed its editorial process and peer review in collaboration with the rest of the editorial team.
The International Weed Genomics Consortium is supported by BASF SE, Bayer AG, Syngenta Ltd, Corteva Agriscience, CropLife International (Global Herbicide Resistance Action Committee), the Foundation for Food and Agriculture Research (Award DSnew-0000000024), and two conference grants from USDA-NIFA (Award numbers 2021–67013-33570 and 2023-67013-38785).
Author information
Authors and affiliations.
Department of Agricultural Biology, Colorado State University, 1177 Campus Delivery, Fort Collins, CO, 80523, USA
Jacob Montgomery, Sarah Morran & Todd A. Gaines
Protecting Crops and the Environment, Rothamsted Research, Harpenden, Hertfordshire, UK
Dana R. MacGregor
Department of Crop, Soil, and Environmental Sciences, Auburn University, Auburn, AL, USA
J. Scott McElroy
Department of Plant and Environmental Sciences, University of Copenhagen, Taastrup, Denmark
Paul Neve & Célia Neto
IFEVA-Conicet-Department of Ecology, University of Buenos Aires, Buenos Aires, Argentina
Martin M. Vila-Aiub & Maria Victoria Sandoval
Department of Ecology, Faculty of Agronomy, University of Buenos Aires, Buenos Aires, Argentina
Analia I. Menéndez
Department of Botany, The University of British Columbia, Vancouver, BC, Canada
Julia M. Kreiner
Institute of Crop Sciences, Zhejiang University, Hangzhou, China
Longjiang Fan
Department of Biology, University of Massachusetts Amherst, Amherst, MA, USA
Ana L. Caicedo
Department of Plant and Wildlife Sciences, Brigham Young University, Provo, UT, USA
Peter J. Maughan
Bayer AG, Weed Control Research, Frankfurt, Germany
Bianca Assis Barbosa Martins, Jagoda Mika, Alberto Collavo & Bodo Peters
Department of Crop Sciences, Federal University of Rio Grande Do Sul, Porto Alegre, Rio Grande Do Sul, Brazil
Aldo Merotto Jr.
Department of Soil and Crop Sciences, Texas A&M University, College Station, TX, USA
Nithya K. Subramanian & Muthukumar V. Bagavathiannan
Department of Plant, Soil and Microbial Sciences, Michigan State University, East Lansing, MI, USA
Luan Cutti & Eric L. Patterson
Department of Agronomy, Kansas State University, Manhattan, KS, USA
Md. Mazharul Islam & Mithila Jugulam
Department of Plant Pathology, Kansas State University, Manhattan, KS, USA
Bikram S. Gill
Crop Protection Discovery and Development, Corteva Agriscience, Indianapolis, IN, USA
Robert Cicchillo, Roger Gast & Neeta Soni
Genome Center of Excellence, Corteva Agriscience, Johnston, IA, USA
Terry R. Wright, Gina Zastrow-Hayes, Gregory May, Kevin Fengler & Victor Llaca
School of Agriculture, Food and Wine, University of Adelaide, Glen Osmond, South Australia, Australia
Jenna M. Malone
Jealott’s Hill International Research Centre, Syngenta Ltd, Bracknell, Berkshire, UK
Deepmala Sehgal, Shiv Shankhar Kaundun & Richard P. Dale
Department of Plant and Soil Sciences, University of Pretoria, Pretoria, South Africa
Barend Juan Vorster
BASF SE, Ludwigshafen Am Rhein, Germany
Jens Lerchl
Department of Crop Sciences, University of Illinois, Urbana, IL, USA
Patrick J. Tranel
Senior Scientist Consultant, Herbicide Resistance Action Committee / CropLife International, Liederbach, Germany
Roland Beffa
School of BioSciences, University of Melbourne, Parkville, VIC, Australia
Alexandre Fournier-Level
You can also search for this author in PubMed Google Scholar
Contributions
JMo and TG conceived and outlined the article. TG, DM, EP, RB, JSM, PJT, MJ wrote grants to obtain funding. MMI, BSG, and MJ performed mitotic chromosome visualization. VL performed sequencing. VL and KF assembled the genomes. LC and ELP annotated the genomes. JMo, SM, DRM, JSM, PN, CN, MV, MVS, AIM, JMK, LF, ALC, PJM, BABM, JMi, AC, MVB, LC, AFL, and ELP wrote the first draft of the article. All authors edited the article and improved the final version.
Corresponding author
Correspondence to Todd A. Gaines .
Ethics declarations
Ethics approval and consent to participate.
Ethical approval is not applicable for this article.
Competing interests
Some authors work for commercial agricultural companies (BASF, Bayer, Corteva Agriscience, or Syngenta) that develop and sell weed control products.
Additional information
Publisher’s note.
Springer Nature remains neutral with regard to jurisdictional claims in published maps and institutional affiliations.
Supplementary Information
13059_2024_3274_moesm1_esm.docx.
Additional file 1. List of completed and in-progress genome assemblies of weed species pollinated by insects (Table S1).
13059_2024_3274_MOESM2_ESM.docx
Additional file 2. Methods and results for visualizing and counting the metaphase chromosomes of hexaploid Avena fatua (Fig S1); diploid Lolium rigidum (Fig S2); tetraploid Phalaris minor (Fig S3); and tetraploid Salsola tragus (Fig S4).
Additional file 3. Review history.
Rights and permissions.
Open Access This article is licensed under a Creative Commons Attribution 4.0 International License, which permits use, sharing, adaptation, distribution and reproduction in any medium or format, as long as you give appropriate credit to the original author(s) and the source, provide a link to the Creative Commons licence, and indicate if changes were made. The images or other third party material in this article are included in the article's Creative Commons licence, unless indicated otherwise in a credit line to the material. If material is not included in the article's Creative Commons licence and your intended use is not permitted by statutory regulation or exceeds the permitted use, you will need to obtain permission directly from the copyright holder. To view a copy of this licence, visit http://creativecommons.org/licenses/by/4.0/ . The Creative Commons Public Domain Dedication waiver ( http://creativecommons.org/publicdomain/zero/1.0/ ) applies to the data made available in this article, unless otherwise stated in a credit line to the data.
Reprints and permissions
About this article
Cite this article.
Montgomery, J., Morran, S., MacGregor, D.R. et al. Current status of community resources and priorities for weed genomics research. Genome Biol 25 , 139 (2024). https://doi.org/10.1186/s13059-024-03274-y
Download citation
Received : 11 July 2023
Accepted : 13 May 2024
Published : 27 May 2024
DOI : https://doi.org/10.1186/s13059-024-03274-y
Share this article
Anyone you share the following link with will be able to read this content:
Sorry, a shareable link is not currently available for this article.
Provided by the Springer Nature SharedIt content-sharing initiative
- Weed science
- Reference genomes
- Rapid adaptation
- Herbicide resistance
- Public resources
Genome Biology
ISSN: 1474-760X
- Submission enquiries: [email protected]
- General enquiries: [email protected]
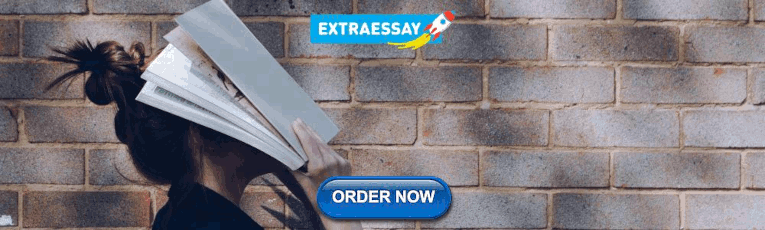
COMMENTS
Importance of Translational Research. In one study of 101 very promising claims of scientific discoveries with unambiguous clinical potential published in major science journals between 1979 and 1983, only five resulted in interventions with licensed clinical use by 2003. 8 Estimates of the median time required for new scientific discoveries to result in successfully completed clinical trials ...
Section edited by Richard J. Ablin and Marcello Maggiolini. The Hot Topics section will publish a limited number of high interest review articles per year, focusing on cutting edge topics from across the breadth of the journal's scope. Articles will cover emerging concepts and points of view or foresight, rather than focusing on comprehensive ...
About the journal. The Journal of Laboratory and Clinical Medicine. In Association with Renji Hospital, Shanghai Jiao Tong University School of Medicine. Translational Research delivers original investigations in the broad fields of laboratory, clinical, and public health research. Published monthly since 1915, it …. View full aims & scope ...
Introduction. Clinical and translational research (CTR) has been experiencing a resurgence since the mid-2000s along with an embracing of team science within the CTR community [1-4].Around this same time, articles questioning or discussing the validity of published research results began to emerge in academic as well as nonacademic publications [5-7].
Translational research involves the application of knowledge gained through basic research to studies that could support the development of new products. For example, translational research in the ...
The term "translational research" was first used in a US national cancer program in the 90's and has since appeared in research programs, research strategies, academic articles and journals ...
Instead, all articles published in Pediatric Research represent translational research and categorized based on methodology: basic translational research, clinical translational research, or ...
Translational medicine is a new way of thinking that connects basic medical research with clinical treatment. Translational medicine drives basic research with clinical treatment, promotes clinical treatment with basic research, and promotes mutual transformation. Translational medicine breaks the barrier between basic research and clinical ...
Translational Research in the Fastest-Growing Population. Kevin P. High, Stephen Kritchevsky, in Principles of Translational Science in Medicine (Second Edition), 2015 Conclusion. Translational research in aging has the potential to influence lifespan and healthspan beyond the boundaries of traditional disease-based research. There are many animal models and human cohorts that are useful in ...
The context of translational research. Translational research has emerged as an important driver to facilitate conversion and increase biomedical productivity (Fishburn, 2013; Van der Laan & Boenink, 2015; Woolf, 2008; Zerhouni, 2003).Translational research is generally understood as a concerted effort to produce new products, services, or treatments from basic research in a rapid manner ...
Translational Issues in Psychological Science ® (TPS) is a critical issues translational journal, with each issue on a different topic representing multiple viewpoints on psychological science.. Each issue of TPS concentrates on a single important, timely, and/or potentially controversial theme in translational science that is of broad interest to scientists, practitioners, and the general ...
Translational research. Translational research (also called translation research, translational science, or, when the context is clear, simply translation) [1] [2] is research aimed at translating (converting) results in basic research into results that directly benefit humans. The term is used in science and technology, especially in biology ...
Many definitions have been given for translational research, but the definition I like best is that it is a systematic effort to convert basic research knowledge into practical applications to enhance human health and well being. Translational research was designed for the medical world. It emerged in response to concern over the long time lag ...
Topic Information. Dear Colleagues, Translational research is one of the most essential but challenging areas of laboratory sciences when it comes to understanding the underlying pathomechanisms of, to search new biomarkers of, as well as to develop novel therapeutics for, neurological and psychiatric disorders.
guo-yuan yang. Med-X Research Institute, School of Biomedical Engineering, Shanghai Jiao Tong University. Shanghai, China. Specialty Chief Editor. Translational Neuroscience.
Translational research promotes the multidirectional and multidisciplinary integration of basic research, patient-oriented research, and population-based research ( Rubio et al., 2010) necessary to inform interventions and policies to eliminate health disparities. It is important that all such research and related interventions and policies are ...
Translational Research publishes original investigations in the broad fields of laboratory, clinical, and public health research. It keeps readers up-to-date on significant biomedical research from all subspecialties of medicine. Starting in 2023, there will be two Co-Editors-in-Chief leading an international and diverse team of editors:
Mapping of terms used in research on P. gangrenosum. A: The co-occurrence network of terms extracted from the title or abstract of at least 40 articles.The colors represent groups of terms that are relatively strongly linked to each other. The size of a term signifies the number of publications related to P. gangrenosum in which the term appeared, and the distance between two terms represents ...
2006 — Volume 148. Formerly known as Journal of Laboratory and Clinical Medicine; Read the latest articles of Translational Research at ScienceDirect.com, Elsevier's leading platform of peer-reviewed scholarly literature.
The Translational Research Program (TRP) is committed to reducing cancer incidence and mortality and improving survival and quality of life for cancer patients. TRP uses advances in basic sciences to develop new approaches for the prevention, diagnosis, and treatment of cancer by fostering interdisciplinary investigations and coordinating the ...
These 5- to 15-minute videos offer an overview and/or practical guidance on a wide variety of research-related topics ranging from study development to dissemination of research results. ... Clinical and Translational Science Institute. Clinical and Translational Research Center. 875 Ellicott Street Buffalo, NY 14203. 716-888-4850 ctsi@buffalo ...
Their preliminary findings, published in Science Translational Medicine, suggest that this sensitive, non-invasive approach could open new avenues for patient monitoring and clinical research, as ...
Translation research has four stages (Table 1): Stage 1, development translation research, studies how a discovery made in a laboratory, field or pilot study or findings of risks, can move into a potential health and safety application to be tested. Stage 2, testing translation research, assesses the value of a new finding, invention, process, training program, or intervention.
The outcomes of each of these domains could include (1) evidence that courses, seminars, workshops, and laboratory experiences offered in the program lead to fulfillment of preestablished competency requirements; (2) evidence of improvement over time in the trainees' knowledge and skills regarding translational research topics and endeavors ...
News Topics. Translational Research. Share: Print page Facebook share Linkedin share X social media share. All News & Events ... Announcements; Translational Research March 14, 2024 Scientists create tools to identify patients with potentially treatable forms of rapidly progressive dementia May 11, 2023 Less myelin content in the brain linked ...
As CTR co-directors, Drs. Goyal and Tarini will lead the hospital's mission to advance translational science, clinical research and community health. Children's National Hospital has appointed two nationally regarded leaders in pediatric research - Monika K. Goyal, M.D., M.S.C.E., and Beth A. Tarini, M.D., M.S., M.B.A. - to head its ...
Survival analyses are statistical methods for the analysis of time-to-event outcomes. 1 An example is time from study entry to death. A competing risk is an event whose occurrence precludes the occurrence of the primary event of interest.
Elizabeth Selvin has been appointed the next director of the Welch Center for Epidemiology and Clinical Research at the Johns Hopkins University, effective May 1. She succeeds Lawrence J. Appel, who led the center for the past 14 years. A collaborative effort between the Johns Hopkins Bloomberg School of Public Health, the School of Medicine, and the School of Nursing, the Welch Center was ...
Weeds are attractive models for basic and applied research due to their impacts on agricultural systems and capacity to swiftly adapt in response to anthropogenic selection pressures. Currently, a lack of genomic information precludes research to elucidate the genetic basis of rapid adaptation for important traits like herbicide resistance and stress tolerance and the effect of evolutionary ...
Abstract. Translational research is a bidirectional process that involves multidisciplinary integration among basic, clinical, practice, population, and policy-based research. The goal of translational research is to speed up scientific discovery into patient and community benefit. This chapter provides an overview of key concepts, methods, and ...