Advertisement
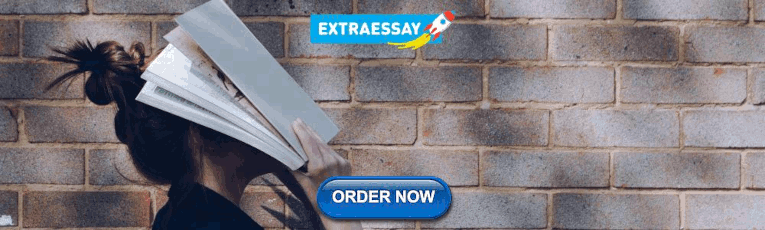
Digital health: trends, opportunities and challenges in medical devices, pharma and bio-technology
- Published: 11 April 2023
- Volume 11 , pages 11–30, ( 2023 )
Cite this article
- Naresh Kasoju ORCID: orcid.org/0000-0002-8700-0729 1 ,
- N. S. Remya ORCID: orcid.org/0000-0003-4068-4147 1 ,
- Renjith Sasi ORCID: orcid.org/0000-0002-6430-2556 1 ,
- S. Sujesh 1 ,
- Biju Soman ORCID: orcid.org/0000-0003-0748-0839 1 ,
- C. Kesavadas ORCID: orcid.org/0000-0003-4914-8666 1 ,
- C. V. Muraleedharan ORCID: orcid.org/0000-0002-8398-0379 1 ,
- P. R. Harikrishna Varma 1 &
- Sanjay Behari 1
10k Accesses
8 Citations
17 Altmetric
Explore all metrics
Digital health interventions refer to the use of digital technology and connected devices to improve health outcomes and healthcare delivery. This includes telemedicine, electronic health records, wearable devices, mobile health applications, and other forms of digital health technology. To this end, several research and developmental activities in various fields are gaining momentum. For instance, in the medical devices sector, several smart biomedical materials and medical devices that are digitally enabled are rapidly being developed and introduced into clinical settings. In the pharma and allied sectors, digital health-focused technologies are widely being used through various stages of drug development, viz. computer-aided drug design, computational modeling for predictive toxicology, and big data analytics for clinical trial management. In the biotechnology and bioengineering fields, investigations are rapidly growing focus on digital health, such as omics biology, synthetic biology, systems biology, big data and personalized medicine. Though digital health-focused innovations are expanding the horizons of health in diverse ways, here the development in the fields of medical devices, pharmaceutical technologies and biotech sectors, with emphasis on trends, opportunities and challenges are reviewed. A perspective on the use of digital health in the Indian context is also included.
Avoid common mistakes on your manuscript.
1 Introduction
Digital health is a rapidly growing field that offers exciting opportunities for innovation and improvement in healthcare delivery. The goal of digital health is to make healthcare more efficient, accessible, and effective, by leveraging the power of digital technology to collect, analyze, store and share health data. Electronic Health Records (EHRs), telemedicine, mobile health apps, wearable devices, the internet of medical things and cutting-edge digital technology constitute digital health. The digital health market has been growing rapidly in recent years and is expected to continue its growth trajectory in the near future. The global digital health market size was valued at approximately US$211 billion in 2022 and is expected to grow at a compound annual growth rate (CAGR) of 18.6% from 2023 to 2030 [ 1 ]. In the Indian context, the digital health market is reported to be about US$12.2 billion in 2023 and is projected to reach US$25.64 billion by 2027 with a CAGR of about 20.4% [ 2 ]. The digital health market is highly fragmented and is characterized by a large number of small and medium-sized enterprises operating in various segments, such as wearable devices, telemedicine, EHRs, and mobile health apps. Major players in the digital health market include Apple, Google, Philips, Medtronic, and Roche, among others [ 3 ].
The growth of the digital health market can be attributed to several factors, including the increasing adoption of smartphones and other digital devices, the growing demand for remote monitoring and telemedicine services, and the increasing focus on the development of digital health solutions to address the challenges posed by the COVID-19 pandemic. Several groups are working on various aspects of digital health, and the number of scientific publications in this area has been growing rapidly in recent years (Fig. 1 ).
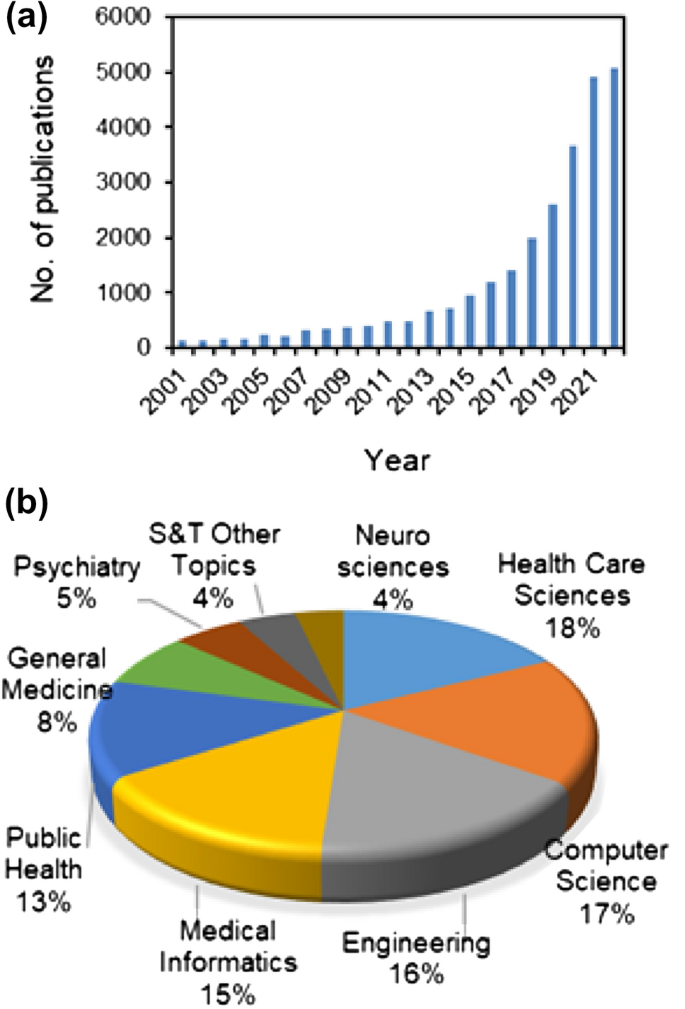
An overview of the publication trends in digital health as available from the web of science ( a ), and details of sectors where much of the research work is being focused ( b )
The medical device sector has seen significant innovations in digital health in recent years. These range from wearable devices, remote monitoring systems, telemedicine devices, and electronic drug dispensing units to smart inhalers. These innovations in the medical device sector have the potential to greatly improve healthcare delivery and patient outcomes by providing more efficient and effective ways to monitor and manage health. Similarly, in the pharma sector, Digital Health Technologies (DHTs) are being used in many ways. Several DHTs are being used to speed up the drug development process through (i) drug design by virtual screening tools, (ii) reducing animal usage by predictive toxicology, and (iii) streamlining clinical trials by digital data management. In the biotechnology and bioengineering front, developments in the field of omics, synthetic and systems biology, big data and precision medicine are leaning towards digital health. This review gives an overview of the trends, opportunities and challenges for digital health innovations in the medical device, pharma and bio-allied fields. Although there are pervious review articles on digital health in general, the current review was the first of its kind covering digital health technologies across the key segments in the health sector i.e. medical devices, pharma and biotechnology.
2 Developments in medical devices and allied technologies toward digital health
2.1 medical devices.
Digital health-focused medical devices are devices that utilize digital technologies to improve health and healthcare. These devices are playing an increasingly important role in improving healthcare delivery by enabling remote patient monitoring, increasing access to medical services, and reducing healthcare costs. They also offer the potential for improved patient outcomes by enabling early detection and intervention in medical conditions. However, there are challenges associated with the use of these devices, such as the need for appropriate regulatory oversight, privacy concerns, and attention to cybersecurity risks. Examples of digital health-focused medical devices are described below.
2.1.1 Wearable devices
Wearable technology has been an active area of research in recent years, with numerous advances. These devices such as smartwatches and fitness trackers monitor various aspects of a person's health, such as heart rate, sleep patterns, and physical activity [ 4 ]. Some wearable devices also have features such as ECG monitoring and fall detection. The latest wearable medical devices global market report underlines that the market would grow from US$22.44 billion (2022) to US$27.37 billion by the end of 2023, with a predicted annual growth rate of 21.9%. It further suggests that the trend will continue with the same CAGR to reach $60.48 billion in 2027. Following are some of the latest trends and developments in this area:
(a) Augmented reality (AR) integrated wearable devices It is an area that has seen significant growth in recent years [ 5 , 6 ]. Researchers are exploring ways to integrate AR technology into wearable devices to create an enhanced user experience. This could include things like displaying information directly on a user's field of vision or providing additional context to the real world.
(b) Artificial Intelligence (AI) integrated wearable devices AI is also being integrated into wearable devices to provide users with more advanced features and capabilities [ 7 ]. For example, wearable devices could use AI to analyze data from various sensors and make predictions about a user's health or provide personalized recommendations.
(c) Energy harvesting wearable devices This is another area of research in wearable technology [ 8 ]. Researchers are developing devices that can generate their power through movement, body heat, or other sources, which would make them more self-sufficient and reduce the need for charging.
A schematic representation of wearable, minimally invasive/implantable devices integrated with DHTs is presented in Fig. 2 .
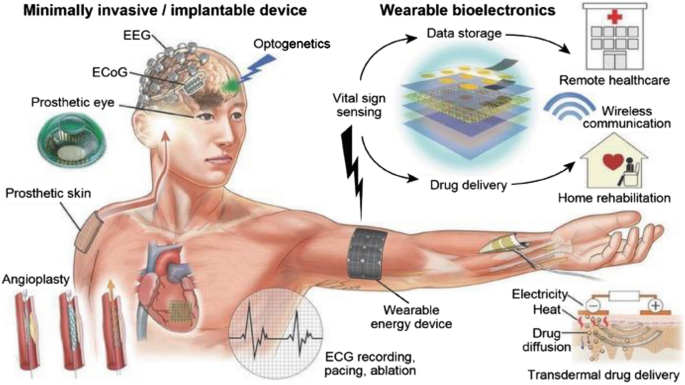
A schematic illustration of an array of wearable, minimally invasive/implantable devices integrated with DHTs. Reproduced with permission from [ 9 ]. © 2016 WILEY‐VCH Verlag GmbH & Co. KGaA, Weinheim
2.1.2 Diagnostic devices
These are devices used for diagnostic purposes, such as glucose meters for diabetic patients, spirometry devices for pulmonary function testing, and portable ultrasound machines. Following are some of the latest trends and developments in this area:
(a) Point-of-care (POC) diagnostics POC testing devices are becoming increasingly popular as they allow for rapid diagnostic testing at the point of care. These devices are designed to be small, portable, and easy to use, making it possible to diagnose a wide range of conditions in a variety of settings [ 10 ].
(b) Non-invasive diagnostics Non-invasive diagnostic devices are being developed to provide a more comfortable and convenient testing experience for patients [ 11 ]. For example, devices that use breath analysis or skin sensors to diagnose conditions are being developed, eliminating the need for invasive procedures such as blood tests.
(c) Nanotechnology-based diagnostics Nanotechnology is being used to develop new diagnostic devices that are more sensitive and efficient [ 12 ]. For example, nanoparticle-based devices are being developed that can detect specific biomarkers in blood and other body fluids, allowing for the early detection of diseases such as cancers.
2.1.3 Therapeutic devices
These are devices used for treatment, such as insulin pumps, implantable cardiac pacemakers, and deep brain stimulation devices. Following are some of the latest trends and developments in this area:
(a) Wearable therapeutics These devices are becoming increasingly popular as they allow for continuous and non-invasive treatment. For example, wearable devices are being developed to deliver electrical stimulation to the brain to treat conditions such as depression or to deliver drugs directly to the site of an injury [ 13 , 14 ].
(b) Non-invasive stimulators Non-invasive stimulation devices, such as transcranial magnetic or electrical stimulators, are being developed to treat a variety of conditions, including depression, anxiety, and chronic pain. These devices use magnetic or electrical fields to stimulate specific regions of the brain, providing a safe and non-invasive alternative to traditional treatments [ 15 ].
(c) Regenerative therapeutics Regenerative medicine is a growing area of research, and therapeutic devices are being developed to support the growth and regeneration of damaged tissue [ 16 ]. For example, devices are being developed to deliver growth factors to the site of an injury, promoting tissue repair and regeneration.
2.1.4 Medical imaging devices
These are devices used for imaging the body, such as X-ray machines, CT scanners, and MRI machines. Following are some of the latest trends and developments in this area:
(a) Artificial Intelligence-enabled classification and detection which is being used to improve the accuracy and efficiency of medical imaging devices [ 17 ]. For example, AI algorithms can be trained to identify patterns in medical images, such as X-rays or CT scans, helping healthcare providers make more informed diagnoses and prognoses.
(b) Medical imaging-assisted customized 3D printing products medical imaging-assisted 3D printing technology is being used to create customized medical devices, such as surgical models, prosthetics and implantable systems [ 18 , 19 ]. This can be particularly useful for patients with complex medical conditions, as 3D printing allows for the creation of devices that are tailored to the individual's specific needs.
(c) Non-Invasive Imaging Invasive imaging modalities are followed for diagnosing complicated medical conditions, such as coronary angiography for diagnosing coronary artery stenosis. To this end, non-invasive imaging devices that use ultrasound or optical imaging to visualize internal organs or tissues are being developed for detecting various conditions, such as coronary artery stenosis, myocardial infarction, liver metastasis, and beyond [ 20 ].
A schematic representation of AI-based medical image detection and analysis in the context of COVID-19 is presented in Fig. 3 .
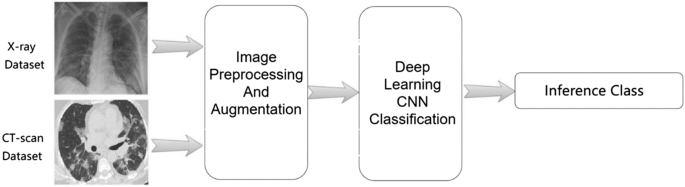
A schematic showing deep learning-based medical image detection and analysis in the context of COVID-19. Reproduced from [ 21 ]. © The Authors 2021
2.1.5 Telemedicine devices
These are devices that enable remote patient monitoring and teleconsultations, such as remote patient monitoring systems, webcams, and handheld devices with cameras and communication capabilities. Following are some of the latest trends and developments in this area.
(a) Wearable telemedicine devices These devices are becoming increasingly popular, as they allow for continuous monitoring of a patient's health status [ 22 ]. These devices can track vital signs, such as heart rate and blood pressure, and transmit the data to healthcare providers for analysis.
(b) Remote diagnostic and intervention tools These tools are being developed to allow healthcare providers to diagnose and attend to conditions remotely [ 11 , 23 ]. For example, some telemedicine devices are equipped with cameras and other tools that allow healthcare providers to examine and intervene as necessary to attend to a patient remotely.
(c) Integration with Electronic Health Records Telemedicine devices are being integrated with EHRs to provide a more comprehensive view of a patient's health status [ 24 , 25 ]. This integration can help healthcare providers make more informed decisions about a patient's treatment plan, as they have access to a patient's complete medical history.
A schematic representation showing various DHT-enabled telemedicine modalities is presented in Fig. 4 .
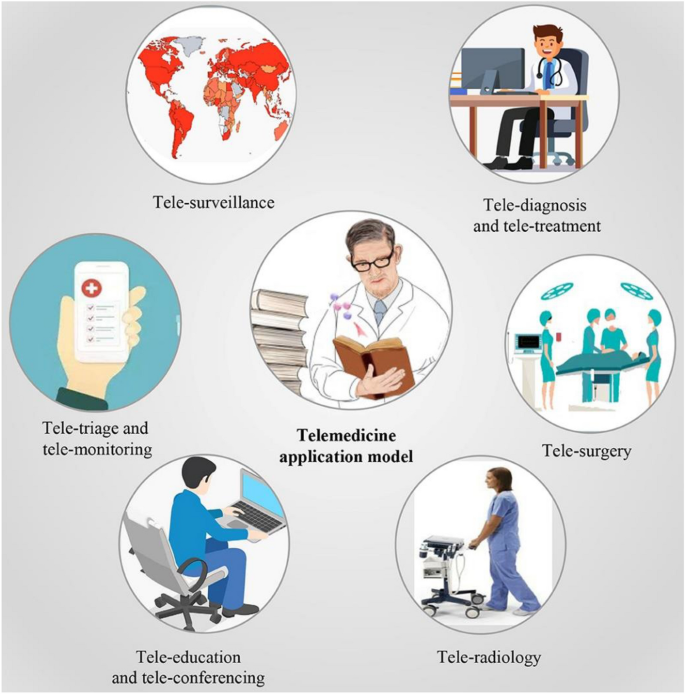
A schematic showing various avenues of digital health technology-enabled telemedicine modalities. Reproduced from [ 26 ]. © The Authors 2021
2.2 Medical materials
As in many other avenues, the advent of digital health paved the way for tremendous development in the field of material science and related research [ 27 ]. Modern material science can contribute smart materials and analytical tools suitable for developing wearable medical devices and sensors required for this purpose. The wearable devices could help in the monitoring of chronic health conditions, therapy, diagnosis, rehabilitation, and tracking of physical activities [ 28 ]. Timely interventions supported by the real-time monitoring of health parameters using wearable devices would save the lives of many. The cloud-based operation of wearable medical devices enables medical professionals to monitor real-time vital parameters and to plan the requirement of physical visits, changes in therapy, and modalities for disease management. A schematic representation showing the work-flow behind cloud-based device performance is presented in Fig. 5 .
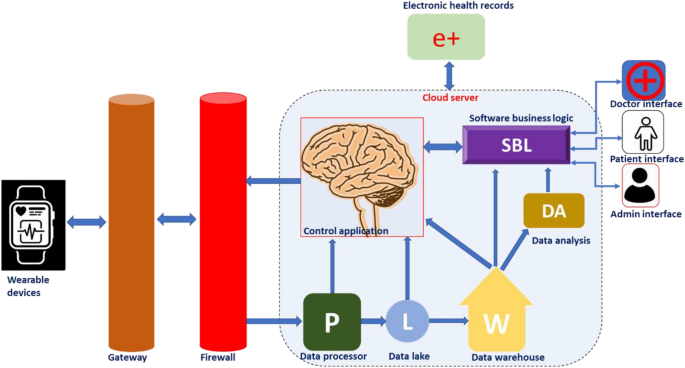
Schematic representation of work flow behind a cloud-based wearable device
The materials used for the fabrication of smart wearable medical devices should be biocompatible, flexible/wearable, lightweight, cost-effective, and smart enough to generate transmittable signals in response to changes in physiological parameters such as arterial pulse, body temperature, humidity, motion, and biomarkers in body fluids. Recognition of signals and their transformation are the two fundamental processes associated with any sensors used in the healthcare sector [ 29 ]. In wearable medical devices, the sensors respond to various parameters such as pressure, strain, temperature, the concentration of biomarkers, etc., and generate transmittable electronic/optical signals. Pressure/strain sensors, humidity/gas sensors, electrochemical sensors, colorimetric sensors, etc., are the major types of wearable sensors employed in the healthcare sector [ 30 ]. Even though many inorganic and metallic materials are available with excellent conductivity and sensing capability, inflexibility hinders their application in wearable devices.
Recently a wide variety of advanced smart materials have been utilized for developing wearable devices for healthcare applications. The most prominent ones are described below.
2.2.1 Ionic liquids
Ionic liquids were frequently used for the development of wearable sensors owing to their flexibility, conductivity, broad electrochemical window, better miscibility, negligible toxicity, and low vapor pressure [ 31 ]. Ionic liquid-based smart materials have been reported for a wide range of healthcare applications.
Wearable strain/pressure sensors: Ionic liquid smart devices could convert mechanical strain into processable and transmissible electrical signals in both resistive and capacitive modes. wearable motion sensors could be suitable for monitoring the elderly or rehabilitating population to assess their progress and to provide intervention as and when required [ 32 ].
Thermal sensors: As ionic liquids and ionic liquid crystals are capable of thermal transitions of their phases; they could be employed for monitoring the body temperatures of patients [ 33 ].
Breathing monitors: Ionic liquid-based wearable strain sensors were also reported to monitor the breathing events of patients with COPD or sleep apnoea. Stomach attachment of IL-based wearable breath rate sensors would provide alarms during dangerous breath variations or apnoea [ 34 ].
Sensors for cardiovascular parameters: Ionic liquid-based wearable devices were reported for ECG and EMG recordings [ 35 ].
Others: Ionic liquid-based devices were reported for monitoring skin humidity and evolved gases [ 36 ], glucose or lactate levels and pH from sweat [ 37 ], and for applications in therapeutics and drug delivery [ 38 ].
2.2.2 Carbon materials
A wide array of carbon nanomaterials like carbon nanotubes (CNTs), graphene-based materials, and carbon black (CB) were exploited for healthcare applications. Low cost, mass production capability, biocompatibility, and good mechanical and conduction behaviors made them suitable for generating smart medical devices. Carbon-based smart devices could be fabricated by a variety of methods such as chemical vapor deposition, drop casting, spin coating, screen or inkjet printing, and vacuum filtration. Their major applications are:
Wearable sensors for strain, pressure, temperature and humidity [ 39 ].
Biosensors for biomarker detection [ 40 ].
Others: bone and cartilage regeneration, Bioimaging, and Breath analysis [ 41 ].
An overview of various applications of DHT-enabled carbon nanomaterials in medical and other allied fields is presented in Fig. 6 .
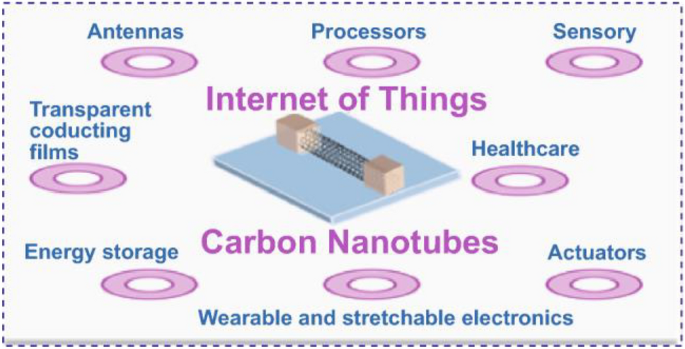
An overview of various applications of DHT-enabled carbon nanomaterials in medical and other allied fields. Reproduced from [ 42 ]. © The Authors 2021
2.2.3 Gold nanomaterials
Gold nanomaterials are known to have better electrical conductivity, mechanical flexibility, biocompatibility, and a wide electrochemical sensing window. Their surfaces could be modified by suitable chemical reactions to improve their electrical and optical behaviors to fine-tune sensing capabilities.
Wearable strain/pressure sensor: Mechanical perturbation on the nano-dimensional gold is converted into a readable electrical signal. They mainly follow a resistance-type, capacitance-type, piezoelectric-type, or triboelectric-type transduction mechanism. Strain/pressure sensors could be applied in soft robotics, human–machine interactions, human motion detection systems, and in health monitoring [ 43 ].
Humidity sensors for human breath analysis: Humidity sensors function on the variation of the impedance values of the membranes with respect to humidity variations [ 44 ].
Others: Gold nanomaterial-based wearable biosensors were reported for various biomarkers, Wearable pH sensors, and bioimaging therapeutics and drug delivery [ 45 ].
In addition to these materials other nano materials, conducting polymers and smart materials were reported to be contributing to the area of digital health [ 46 ]. Thus, material science research flourished extensively due to the arrival of digital health platforms. In addition to material science research, the analytical modalities were also influenced by the rapid development of digital health. NIR and Raman-based non-invasive disease monitoring strategies were reported for the detection of disease conditions and measuring vital parameters [ 47 , 48 ]
3 Developments in pharma and allied areas toward digital health
The tremendous expansion of DHTs at both customer and professional levels has opened a better arena for the effective utilization of digital resources for the benefit of human welfare. In this context, DHTs are playing an increasingly important role in the delivery of pharmaceutical care. Despite the widespread acceptance of personalized technologies in pharma health care, the DHT system is not comprehensively reviewed in terms of drug discovery and development. Drug discovery and development is a complex and multi-step process that involves multiple stages generally taking a time frame of 10–12 years [ 49 ]. The following is a general flowchart that outlines the process of drug discovery:
Target identification and validation: In this stage, researchers identify and validate a biological target (e.g., a protein, gene, or pathway) that is involved in the disease process. They use various high-throughput screening techniques to identify the small molecule or biological entities (hits) that modulate the activity of the target and have potential therapeutic effects [ 50 ].
Lead optimization: In the optimization stage, the researchers optimize the potency, selectivity, pharmacokinetics, and pharmacodynamics of the lead compounds to produce lead candidates [ 51 ].
Pre-clinical evaluation: This is the stage where the researcher conducts a series of in vitro and in vivo studies to evaluate the safety, efficacy, pharmacokinetics, and pharmacodynamics of the lead candidates.
Clinical trials: Lead candidates that have passed preclinical testing are then tested in human clinical trials to evaluate their safety and efficacy in a larger population.
Regulatory approval: If the clinical trials are successful, the drug is then submitted to regulatory agencies for approval.
Marketing and sales: only after the drug gets approved, it can be manufactured and marketed for therapeutic use.
Post-market surveillance: This will be an indefinite process making the regulators monitor the efficacy and safety of the drug throughout its lifetime.
In this context, digital technologies are playing an increasingly important role in the development of new drugs. Some of the key ways that digital technologies are being used in drug development are presented in Fig. 7 and are detailed in the following sections.
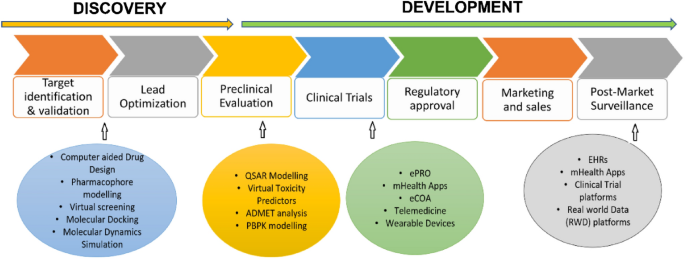
An overview of applications of digital health technologies in drug discovery and development
3.1 Drug design
Computer-aided drug design (CADD) is the process of creating new drugs based on a thorough understanding of the biological target and its interaction with potential drugs [ 52 ]. It is a multi-disciplinary field that combines knowledge from chemistry, biology, pharmacology, and computational modeling to develop new drugs. There are several in silico approaches in practice for computer-aided drug design. This includes,
Pharmacophore modeling It is a computational approach used to predict the molecular features that are responsible for a molecule's biological activity. This is a useful tool for drug discovery and design, as it allows researchers to identify key structural features that are important for a molecule's interaction with its target protein, and to design new molecules that are likely to have similar activities. There are two types of pharmacophore modeling. (a) Ligand-based drug design This approach is based on the structural information of the active ligands that bind to the target. In a study by Kist et al. [ 53 ] by employing a ligand-based drug design approach, novel potential inhibitors of the mTor pathway were identified as having comparable or better properties to that of the classic drug rapamycin. (b) Structure-based drug design This strategy uses the three-dimensional structure of the biological target to design drugs that fit into specific pockets or active sites on the target, effectively blocking its activity. An example of a structure-based drug design strategy is reported in the development of 5 LOX inhibitors, a therapeutic target for asthma as well as other inflammatory diseases [ 54 ]. Catalyst software package, LigandScout, MOE (Molecular Operating Environment, Schrodingers Maestro, PyRx are some of the software packages used to generate pharmacophore models.
Drug target fishing This is a computational approach that is used to identify potential drug targets for a particular disease or biological process. And the goal of drug target fishing is to find proteins or other molecular targets that are likely to be involved in the disease or process of interest and to design drugs that can interact with these targets in a specific way to produce a therapeutic effect [ 55 ]. Different approaches could be employed for drug target identification that includes (i) homology modeling: comparing the structure of a protein of interest to the structures of other related proteins that are already known targets and identifying conserved regions in the protein structure that could serve as a potential target [ 56 ], (ii) bioinformatics: analyzing biological data, such as genomic sequences, transcriptomic data, and protein–protein interaction data, to identify potential drug targets [ 57 ]. (iii) systems biology: studying the complex interactions between different biological components in a particular disease or process [ 58 ] and (iv) High-Throughput screening [ 59 ]. The experimental setup in this context is the molecular docking and molecular dynamics (MD) simulation. There are several widely used software programs available for molecular docking, including AutoDock, Glide, Leadit, and eHiTS. There are several widely used software programs available for molecular dynamics simulation, including GROMACS, AMBER, NAMD, CHARMM, and LAMMPS that have wide applications in drug discovery.
3.2 Pre-clinical research
Pre-clinical research in drug development is a phase where a variety of experiments are conducted to assess the safety and efficacy of a new drug candidate. These may include toxicity studies to determine the potential for harmful effects, pharmacology studies to evaluate the drug’s interactions with the body, and efficacy studies to determine the potential therapeutic benefits of the drug. They involve animal models and the administration of the drugs to animals to assess any adverse effects and determine the optimal dosing regimen. Animal testing has been used for decades to evaluate the safety and efficacy of new drug candidates, but there is growing concern about the ethical and scientific limitations of this approach [ 60 ]. As a result, there is a growing interest in developing alternative methods to animal testing. In silico modeling of biological systems is one such approach that could be employed as an alternative to animal testing. The computer simulations of biological systems predict the behavior of a particular biological system, and hence, are used to evaluate the safety and efficacy of potential new drugs. In silico models can also be used to analyze large amounts of data, such as gene expression data or proteomics data generated following the interaction of the drug with the biological system. By using AI and Machine Learning (ML) algorithms, these models can identify patterns in the data that would not be easily noticeable by a human researcher, providing new insights into the biological mechanisms underlying disease and thereby helping in the appropriate intervention strategies. The following are the commonly used tools in predicting safety and toxicity in pre-clinical research.
QSAR (Quantitative Structure–Activity Relationship) models These are computational tools that are used to predict the biological activity of a chemical compound based on its molecular structure [ 61 ]. ML algorithms, such as artificial neural networks, decision trees, and support vector machines, are then used to identify relationships between molecular descriptors and biological activities [ 62 ]. Several software programs can be used for QSAR modeling, including KNIME, Pipeline Pilot, MOE (Molecular Operating Environment), OChem and R.
Virtual Toxicity Predictors (VTPs) Unlike QSAR models, virtual toxicity predictor software tools use molecular modeling and simulation to predict the toxicity of a potential new treatment based on its molecular structure. QSAR models typically provide a quantitative prediction of the toxicity of a chemical compound, while VTPs can provide more detailed information about the potential toxicity mechanisms [ 63 ]. Some of the popular software programs used for virtual toxicity prediction include ToxCast, Toxtree, VEGA (Virtual Expert System for Toxicity Assessment), OSIRIS, Leadscope and eTOXlab.
ADMET (Absorption, Distribution, Metabolism, Excretion and Toxicity) analysis It helps in understanding the pharmacokinetics and pharmacodynamics of a drug and is a crucial step in the drug discovery process [ 64 ]. There are several software programs available for ADMET analysis, including both commercial and open-source options. Some popular choices include Schrodinger, Simulations Plus, OpenEye, Pipeline Pilot, Molsoft ICM-Pro, SwissADME, DEREK. However, the choice of software will depend on the specific needs of the user and the type of ADMET analysis being performed.
PBPK (Physiologically-Based Pharmacokinetic) modeling This type of modeling takes into account the anatomy and physiology of the body to simulate the distribution and elimination of drugs. The main goal of PBPK modeling is to predict how a drug will behave in the body based on the known physiological properties of the drug and the individual being treated.[ 65 ]. Examples of software available for PBPK modeling include SimCyp GastroPlus, PK-Sim, ADAPT II, PKQuest, MCSim.
3.3 Clinical trials
The primary goal of a clinical trial is to determine if a new drug is effective in treating a specific medical condition and if it is safe for human use. Clinical trials are usually conducted in three phases, each of which provides increasing amounts of information about the drug's safety and effectiveness. Phase 1 trials typically involve a small number of healthy volunteers and are designed to test the drug's safety and identify any side effects. Phase 2 trials involve a larger number of patients with the specific medical condition the drug is intended to treat. These trials are designed to test the drug's effectiveness and gather additional information about its safety. Phase 3 trials involve an even larger number of patients and are the final stage of testing before a drug is submitted for approval by regulatory agencies [ 66 ]. These trials are designed to provide a more complete picture of the drug's benefits and risks and to confirm its effectiveness.
The DHTs are rapidly changing the way clinical trials are conducted. They are being used to streamline the clinical trial process, allowing for faster and more efficient trials[ 67 ] Here are some examples of DHTs used in clinical trials:
Electronic Patient-Reported Outcomes (ePRO) ePRO is a digital tool that allows patients to report symptoms, side effects, and other outcomes directly to the study team. This technology can help improve patient compliance and reduce the need for in-person visits [ 68 ].
Mobile Health (mHealth) Applications mHealth applications can be used to collect data from patients, provide education and support, and monitor health status. This technology can help increase patient engagement and improve data quality [ 69 ].
Electronic Clinical Outcome Assessments (eCOA) eCOA is a digital tool that allows patients to self-report outcomes, such as quality of life, using a smartphone or tablet. This technology can help reduce the burden on patients and improve data quality [ 70 ].
Telemedicine Telemedicine technology, such as video conferencing, can be used to conduct virtual visits with patients. This technology can help reduce the need for in-person visits, increase patient convenience, and improve patient engagement [ 71 ].
Wearable Devices Wearable devices, such as smartwatches and fitness trackers, can be used to collect data on physical activity, sleep patterns, and other health metrics. This technology can help improve data quality and increase patient engagement [ 72 ].
3.4 Post-market surveillance
DHTs have revolutionized the way drugs are monitored after they have been approved and entered the market. In the past, post-market surveillance of drugs relied heavily on passive systems, where healthcare professionals and patients reported adverse events or side effects. However, with the advent of DHTs, this process has become more proactive and efficient. Some examples of DHTs used in post-market surveillance of drugs include:
Electronic health records EHRs provide a centralized platform for healthcare professionals to report and track adverse events associated with drugs. This information can then be analyzed to identify potential safety issues with a drug [ 73 ].
Mobile health (mHealth) applications mHealth apps allow patients to easily report adverse events or side effects from their smartphones. This provides a more direct and convenient way for patients to report issues, which can lead to quicker identification of safety concerns [ 74 ].
Clinical trials platforms Clinical trial platforms have become increasingly digital, allowing for real-time monitoring of drug safety during the clinical trial phase. This information can then be used in post-market surveillance to identify potential safety issues with a drug [ 75 ].
Real-world data (RWD) platforms RWD platforms gather and analyze data from a variety of sources, including EHRs, claims data, and patient-generated data, to provide a more complete picture of a drug's safety profile. This information can be used to identify potential safety issues and monitor the effectiveness of drugs in real-world settings [ 76 ].
Overall, digital technologies are playing a critical role in drug discovery and development, helping to improve the speed, efficiency, and accuracy of the drug development process. This is leading to the discovery of new treatments for a wide range of diseases and conditions, improving patient outcomes and transforming the healthcare industry.
4 Developments in bio and allied technologies toward digital health
Digital health in biotechnology and bioengineering refers to the use of digital technologies to develop and improve biotechnology and bioengineering products and applications. Digital health is playing a critical role in these fields by enabling the development of more sophisticated models of biological systems and by facilitating the optimization of bio-based healthcare product design and manufacture. Following are some of the key areas where the innovation-driven science and technological advances can transform digital health worldwide.
4.1 Omics biology
Omics is a field of study in medicine that encompasses various sub-disciplines such as genomics, transcriptomics, proteomics, metabolomics, and epigenomics. The goal of omics is to understand the underlying mechanisms of biological processes and diseases by looking at the collective behavior of all the molecules involved, such as genes, proteins, and metabolites. By combining data from these various omics disciplines, researchers can gain a more comprehensive understanding of the molecular basis of health and disease, which may lead to the development of new diagnostic tools, therapies, and personalized medicine approaches.
Omics technologies are also playing an increasingly important role in digital health, where they can be leveraged to improve the accuracy and precision of health assessments, diagnoses, and treatments. Following are a few examples of how Omics technologies can aid in digital health.
(a) Personalized medicine Omics data can be used to predict an individual's risk for developing certain diseases, monitor their health status over time, and tailor treatments to their unique genetic profile [ 77 ].
(b) Predictive analytics Predictive models based on omics data can be used to identify individuals who are at high risk for a disease, such as cancer, and to monitor their health status over time, allowing for early intervention and improved outcomes [ 78 ].
(c) Clinical decision support Clinical decision support systems that incorporate omics data can provide healthcare providers with real-time information and recommendations to help them make more informed treatment decisions for their patients [ 79 ].
(d) Omics data collecting medical devices medical devices that collect omics data, such as continuous glucose monitoring systems, can be used to monitor a patient's health status and to provide early warning signs of potential health issues.
(e) Omics data integrated telemedicine platforms that incorporate omics data can provide remote healthcare services, such as virtual consultations, to individuals in remote or underserved communities, improving access to care and outcomes [ 80 ].
A schematic representation showing omics-based DHTs towards personalized medicine is presented in Fig. 8 .
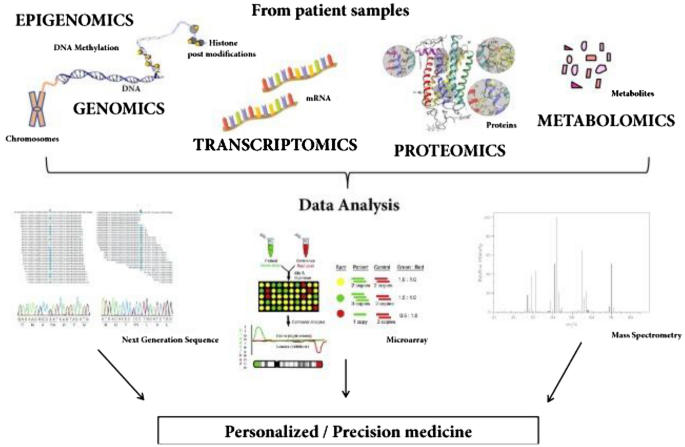
A schematic showing potential applications of omics-based digital health technologies toward personalized medicine. Reproduced from [ 81 ]. © The Authors 2018
4.2 Big data analytics
Big data refers to extremely large and complex data sets that are generated from various sources, including EHRs, medical imaging, genetic sequencing, and other sources. The market size for big data in digital health has been growing rapidly in recent years, driven by the increasing adoption of DHTs and the growing demand for data-driven decision-making in healthcare. According to market research, the global big data in digital health market was valued at approximately US$ 39.7 billion in 2022 and is expected to grow at a CAGR of 19.2% from 2022 to 2032 [ 82 ]. However, the use of big data in medicine also raises concerns about privacy, security, and the ethics of data collection and analysis. It is important to address these concerns and ensure that the benefits of big data are maximized while minimizing its risks.
Big data plays a critical role in digital health by providing the vast amounts of information that are needed to drive innovation and improve patient outcomes.
(a) Big electronic health records data analytics One of the main ways that big data is used in digital health is through the analysis of EHRs and other sources of health-related data [ 83 ]. EHRs contain a vast amount of patient information, including demographic data, medical history, lab results, and other information. By analyzing this data, healthcare providers can gain insights into patient populations and identify trends and patterns that can inform decision-making and improve patient care.
(b) Big wearable devices data analytics big data is used in digital health through the analysis of data generated by wearable devices and other DHTs [ 84 ]. These devices generate vast amounts of data, including information about physical activity, sleep patterns, and other health-related metrics. This data can be used to track health status, monitor disease progression, and inform treatment plans.
(c) Big omics data analytics Another way that big data is used in medicine is through the analysis of genetic data [ 85 ]. Advances in genetic sequencing technologies have enabled the rapid and cost-effective generation of large amounts of genetic data, which can be used to identify the genetic basis of diseases and inform the development of personalized medicine.
(d) Big imaging data analytics Big data is also being used in medical imaging to improve diagnosis and treatment [ 86 ]. For example, advanced algorithms can be used to analyze medical images to identify patterns and anomalies that may indicate disease. This information can then be used to inform diagnosis and treatment planning.
(e) Big data and predictive analytics Big data is also being used in digital health to develop predictive models and algorithms that can improve health outcomes. For example, ML algorithms can be trained on large data sets to identify patterns and relationships that can inform decision-making and improve disease management [ 87 ].
4.3 Personalized/precision medicine
Personalized medicine, also known as precision medicine, is a medical approach that takes into account individual differences in genes, environment, and lifestyle to develop a customized approach to healthcare [ 88 ]. The goal of personalized medicine is to provide the right treatment, at the right time, for the right patient. In traditional medicine, treatments are often based on a "one-size-fits-all" approach, which does not take into account the unique differences between individuals. However, with the advancement of genomic technologies and the increasing availability of patient data, it is now possible to tailor treatments to the specific needs of each patient. It is therefore important to note that personalized medicine is still in its early stages of development, and more research is needed to fully realize its potential.
Several DHTs are playing a critical role in personalized medicine viz. EHRs, telemedicine tools, wearable devices, big data analytics, additive manufacturing, AI/ML-based algorithms, and several personalized mobile apps. These personalized DHTs are playing an increasingly important role in precision medicine, providing healthcare providers with the tools they need to deliver more effective and efficient care to patients. Examples of personalized medicine include:
(a) Precision or personalized care Precision oncology is a type of personalized medicine that uses genetic information to tailor cancer treatments to the specific needs of each patient [ 89 ]. Precision psychiatry is a type of personalized medicine that uses genetic information to tailor psychiatric treatments to the specific needs of each patient [ 90 ].
(b) Precision or personalized drug dosage Personalized drug therapy is a type of personalized medicine that uses genetic information to determine the most effective drug for a particular patient [ 91 ].
(c) Precision surgical models The use of digital technologies such as computer-aided designing and manufacturing helps in scanning the defect site and manufacturing a surgical model utilizing 3D printing for enabling the surgeons to plan the surgery effectively and efficiently [ 92 ].
(d) Personalized regenerative therapies Advanced tissue engineering and regenerative medicine technologies such as 3D bioprinting help in bio-fabricating a tissue that is not only biocompatible but also fits precisely to the defect size of the patient [ 93 ].
(e) Precision public health Proactive use of technology brings in new avenues to address many age-old public health issues. Optimal use of geographic information systems (GIS) and other spatiotemporal analysis will help in more precise and timely field-level interventions, which are crucial in the early detection and control of infectious diseases. This is well documented in the control strategies of the recent Zika outbreak in the USA. In Florida, only two small counties were to be put under lockdown, that too for a short while, to arrest the spread of Zika in 2016 [ 94 ]. The predict and prevent framework is a futuristic method to address the burgeoning problem of non-communicable diseases through precision public health. The use of Electronic medical Support for Public health (ESP) and its visualization platform Riscape helps in long-term follow-up and real-time intervention in the surveillance of NCDs [ 95 ].
A schematic representation showing DHT-enabled personalized medicine is presented in Fig. 9 .
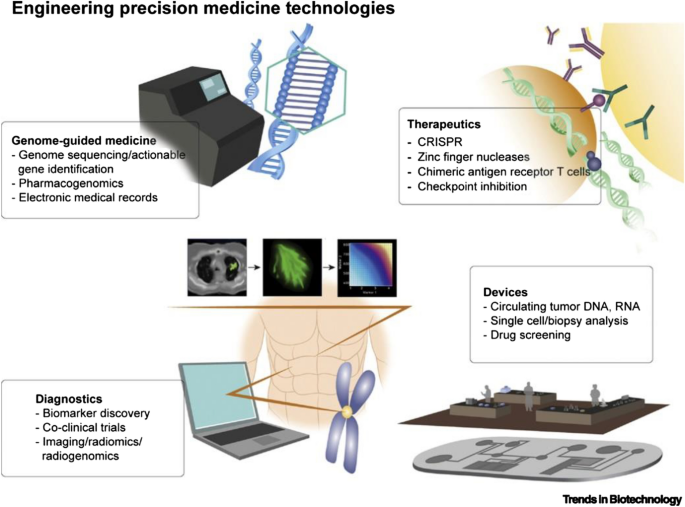
A schematic showing integration of several technologies toward personalized/precision digital health. Reproduced with permission from [ 88 ]. © 2019 Elsevier Ltd
4.4 Synthetic biology
Synthetic biology is a multidisciplinary field that combines biology and engineering to design and construct novel biological systems for various applications [ 96 ]. It involves the manipulation of genetic material and metabolic pathways in living organisms to create new functions or modify existing ones. In synthetic biology, researchers use a combination of molecular biology techniques, computational modeling, and engineering principles to design, build, and test biological systems. These systems can be used in a wide range of applications, including the creation of new medicines, the development of biosensors, and beyond. One of the key features of synthetic biology is the use of standard biological parts, such as genes and regulatory elements, that can be combined and reused to create complex biological systems. This modular approach allows for rapid design and testing, as well as the potential for large-scale deployment of these systems. Synthetic biology has the potential to revolutionize many areas of biotechnology, medicine, and beyond, but it also requires careful consideration and oversight to ensure its safe and responsible development.
Synthetic biology has the potential to play a significant role in digital health [ 97 ]. One example of how synthetic biology can be used in digital health is the development of biosensors [ 98 ]. Biosensors are devices that use biological components, such as enzymes or antibodies, to detect specific substances. Synthetic biology can be used to design and construct biosensors that are specific for certain biomarkers, such as glucose or cholesterol, which can be used for continuous monitoring of health status. The results can be transmitted wirelessly to a digital platform for analysis and interpretation, enabling remote monitoring and disease management. Another example is the use of synthetic biology in the development of personalized medicine [ 99 ]. By using synthetic biology to design and engineer cells, researchers can create new therapeutic interventions that are tailored to a specific individual's needs [ 100 ]. For example, synthetic biology can be used to create cells that produce a specific protein or to correct genetic mutations that cause disease. These cells can then be monitored and controlled using digital technologies, enabling real-time monitoring of treatment efficacy and enabling adjustments to the therapy as needed. Overall, synthetic biology has the potential to revolutionize digital health by enabling the development of new technologies that can be integrated into digital platforms to improve health and healthcare.
4.5 Systems biology
Systems biology is an interdisciplinary field of study that aims to understand the complex relationships between the components of biological systems, such as cells, tissues, and organs [ 101 ]. It seeks to understand how these systems interact and function as a whole, rather than simply focusing on individual components in isolation. Systems biology approaches biological systems from a holistic perspective, using computational and mathematical models to simulate the interactions between different components and to predict the behavior of the system as a whole. It also incorporates high-throughput data from various sources, such as genomics, proteomics, and metabolomics, to create a comprehensive view of the system. One of the key goals of systems biology is to understand the underlying mechanisms of disease and to develop new therapeutic strategies that target the root causes of diseases, rather than just their symptoms. It also seeks to improve our understanding of the interactions between different components of the body and how they contribute to health and disease. In addition, systems biology is playing an important role in the development of personalized medicine, as it provides a framework for integrating patient-specific data and generating personalized models of disease.
Systems biology can play a critical role in digital health in several ways as follows. (a) Predictive Modelling: Systems biology can be used to build predictive models of disease, which can help healthcare providers to identify individuals at risk of developing certain conditions and to develop targeted prevention strategies [ 102 ]. (b) Clinical Decision Support: Systems biology can be used to develop clinical decision support systems, which can help healthcare providers to make more informed treatment decisions based on the latest scientific evidence and patient-specific data [ 103 ]. (c) Clinical Trial Design: Systems biology can be used to inform the design of clinical trials, by helping to identify the most promising therapeutic targets and to predict the outcomes of different treatment strategies [ 104 ]. (d) Data Integration and Management: Systems biology can be used to integrate and manage large amounts of patient data, including genomic, proteomic, and clinical data, to create a comprehensive view of the patient and to inform the development of personalized treatment plans [ 105 ]. (e) Monitoring and Evaluation: Systems biology can be used to monitor the effectiveness of treatments and to evaluate the impact of treatments on patient outcomes [ 106 ]. Overall, systems biology offers a framework for integrating and analyzing large amounts of patient data and for developing personalized models of disease, which can inform the development of more effective and efficient treatment strategies.
A schematic representation showing DHTs integrated systems biology approaches toward effective clinical decisions are presented in Fig. 10 .
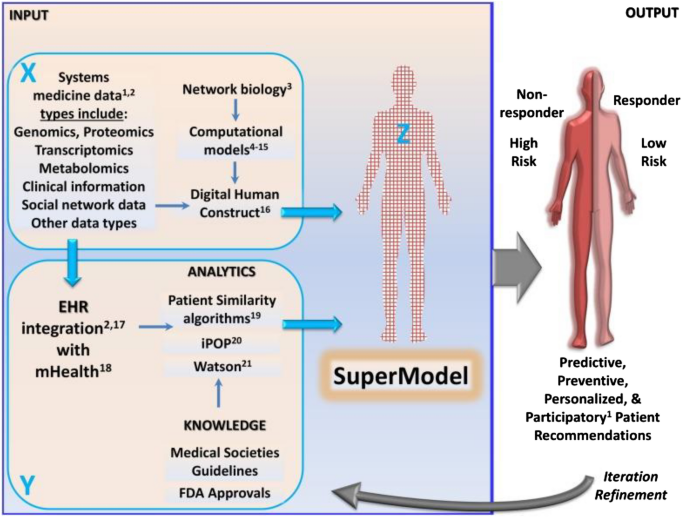
A schematic showing a systems biology approach toward developing super models for effective clinical decisions. Reproduced from [ 107 ]. © The Authors 2015
5 Challenges associated with digital health technologies
With opportunities comes risks, and the same is true for the DHTs that present several challenges along with their opportunities [ 108 ].
(a) Privacy, security and ethical concerns With the increasing growth of mobile-based health apps and connected health systems, much the data including the personal information of patients is being collected. For instance, privacy and security are major concerns in digital health, with the sensitive nature of health data, making it a prime target for hackers. Also, DHTs had several ethical issues including the question of who owns the data.
(b) Interoperability This is another major challenge in digital health, with different digital health systems not being able to communicate with each other effectively. This makes it difficult for healthcare providers to access and share patient data, which can negatively impact patient outcomes.
(c) Regulatory framework Regulation is yet another bottleneck in digital health, with different countries having different regulations and guidelines for DHTs.
(d) Public awareness As many healthcare providers and patients are resistant to change and not fully understanding the benefits of DHTs, creating awareness about its benefits and risks is necessary for ensuring that DHTs are accepted by the general population.
(e) Legislative issues The laws of the land should appreciate the newer trends in science and technology for their optimal use. For example, vagueness in the legal validity of digital prescriptions was a major hurdle in the update of telemedicine in India before the hurried enactment of the Telemedicine Practice Guidelines in 2020, in the wake of the Covid-19 pandemic [ 109 , 110 ].
6 Digital health—an Indian perspective
6.1 digital health—initiatives from the government of india.
Digital health is a rapidly growing field that involves the use of digital technologies to improve health and healthcare delivery in India [ 111 ]. In recent years, there has been a significant investment in digital health infrastructure and initiatives, and a tremendous increase in the use of DHTs by both healthcare providers and patients in India. Examples of digital health initiatives in India include:
(a) Telemedicine Telemedicine services are widely available in India and are being used to provide remote consultations and support to patients in rural and underserved areas [ 112 ].
(b) Electronic health records Several hospitals have initiated programs to establish EHRs for all their clients, and even a comprehensive nationwide EHR platform is under consideration for the storage and sharing of patient health information between healthcare providers [ 113 ].
(c) mHealth The use of mobile health technologies, such as mobile apps and SMS-based services, is widespread in India and is being used to deliver health information and services to patients [ 114 ].
(d) Digital health marketplaces Digital health marketplaces, such as online pharmacies and telemedicine platforms, are becoming increasingly popular in India and are providing patients with access to a wide range of health products and services [ 115 ].
(e) Artificial intelligence in healthcare AI is being used in various applications in the Indian healthcare system, such as in radiology, oncology, and cardiology, to improve diagnosis and treatment [ 116 ].
Several institutions in India are working on digital health, including (a) Indian Council of Medical Research (ICMR): The ICMR is the main body responsible for promoting and coordinating biomedical research in India and has been involved in several digital health initiatives. (b) Apollo Hospitals: Apollo Hospitals is one of the largest healthcare groups in India and is a pioneer in the use of DHTs, including telemedicine and EHRs. (c) Tata Consultancy Services (TCS): TCS is a leading technology and consulting company in India and is involved in several digital health initiatives, including the development of EHRs and telemedicine solutions. (d) MedTech Zone: MedTech Zone is a digital health accelerator program in India that supports the development of early-stage digital health start-ups. (e) AI in Healthcare India: AI in Healthcare India is a non-profit organization that promotes the use of AI in healthcare in India and provides a platform for the exchange of ideas and knowledge on AI in healthcare. These are just a few of the many institutions in India that are involved in digital health initiatives.
Several companies in India are working on digital health, including (a) Practo and Doctor on Call: Practo and Doctor on Call are prominent digital health companies in India offering online doctor appointments including remote consultations and support to patients. (b) NetMeds, Tata 1 mg, Medlife and PharmEasy: these are prominent online platforms for ordering medicines and booking diagnostic tests. (c) HealthKart and HealthifyMe: these are some prominent digital health platforms in India that provide health supplements, and zpersonalized health and wellness coaching. (d) GoQii: GoQii is a digital health platform in India that provides personalized health and wellness coaching and wearable fitness trackers. (e) Besides, several small to medium scale digital health services offer online diagnostic services. These are just a few of the many companies in India that are involved in digital health initiatives. The Indian digital health market is rapidly growing, and many more companies and start-ups are entering the market.
The National Health Authority of the Government of India supports digital health through several schemes. Some of the prominent schemes include (a) Digital India: Digital India is a government initiative in India that aims to transform India into a digitally empowered society and knowledge economy. The initiative includes several components related to digital health, including CoWin and Arogya Sethu ( https://digitalindia.gov.in/ ). (b) e-Health & Telemedicine: various Information & Communication Technologies (ICT)-enabled initiatives are undertaken for improving the efficiency and effectiveness of the public healthcare system ( https://main.mohfw.gov.in/Organisation/departments-health-and-family-welfare/e-Health-Telemedicine ). (c) Ayushman Bharat Digital Mission (ABDM ): through this mission, the Govt. of India aims to develop the backbone necessary to support the integrated digital health infrastructure of the country ( https://abdm.gov.in/ ). (d) National Digital Health Mission (NDHM): The NDHM is a government-led health mission in India that aims to provide universal health coverage to all the citizens in the country through digital technologies ( https://www.makeinindia.com/national-digital-health-mission ). (e) National Health Stack (NHS): The program aims to facilitate the collection of comprehensive healthcare data to aid in policymaking, allocation of resources and identification of needy populations for health schemes. These are just a few of the many government schemes and it is seen that the Indian government is committed to promoting and supporting digital health initiatives in the country. A flyer released by Govt. of India on NDHM is presented in Fig. 11 .
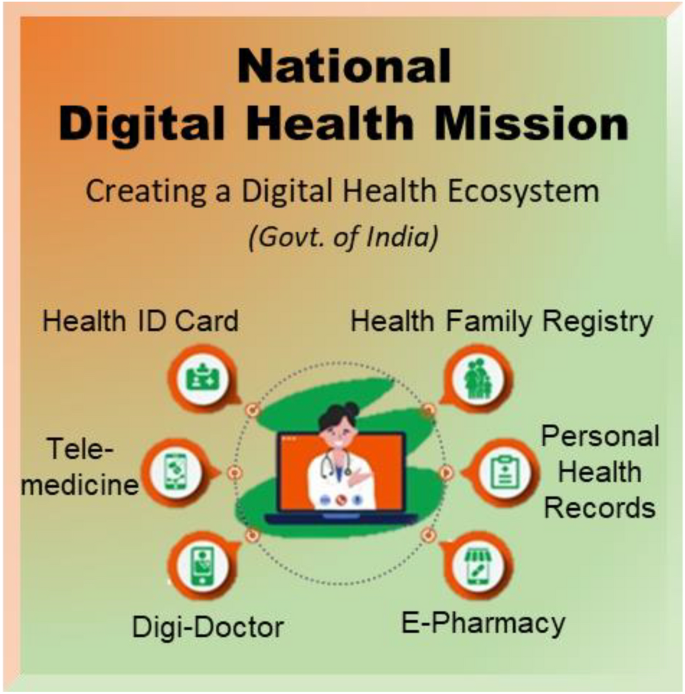
A schematic representation showing various components of the National Digital Health Mission initiative by the Government of India. Adopted with permission from [ 117 ]. © 2023 Sanskriti IAS
Despite the significant opportunities and progress in digital health in India, several challenges need to be addressed, including the need for robust privacy and security measures, the need for greater investment in digital health infrastructure, and the need for greater training and capacity building for healthcare providers.
6.2 Digital health—initiatives from the state of Kerala (India)
Kerala is at the forefront of implementing EHRs for its population. Well before the era of the Individual Health ID of the Ayushman Digital Health Mission, Govt. of Kerala launched its ambitious eHealth Kerala project to create EHRs for all of its citizens in 2016. Though not perfect, it has added to the impetus of digitalization of the health sector in Kerala, thanks to the earlier implementation of the District Health System software (DHIS2) in all the 1000-plus public health institutions in the state.
The health workers in Kerala are familiar with digital health tools, and many public health centers use electronic medical records. However, the use of data for decision-making is not yet a norm in the health system, nor the public health area. Reasons for this low use of information are many, the lack of a clear data policy on who can have access to the data at various levels is a major one.
The state could take advantage of the e-Sanjeevani telemedicine platform during the Covid-19 pandemic to cater to the healthcare needs of its population. In the current e-Health Kerala project, there is a facility to do telemedicine consultations within the regular consultation hours. A few institutions in Kerala, like the Regional Cancer Centre (RCC) and SCTIMST, are going ahead with the doctor-to-doctor e-Sanjeevani consultations.
6.3 Digital health—initiatives from SCTIMST, Trivandrum (India)
Sree Chitra Tirunal Institute for Medical Sciences and Technology (SCTIMST) is an Institution of National Importance under the Department of Science and Technology, Govt. of India. The institute is known for its high-quality advanced treatment of cardiac and neurological disorders, indigenous development of technologies for biomedical devices and public health training and research. The Institute has three wings—the Hospital, Biomedical Technology Wing and the Achutha Menon Centre for Health Science Studies (AMCHSS). The institute is proactive in catching up with the latest technologies in the field including DHTs.
In the clinical scenario, the medical wing of the institute has a custom-built electronic medical record system for its clinical services, entirely created by the in-house computer division. In recent years, the institute has incorporated newer standards, including the SNOMED-CT coding for the diagnostic fields. The anonymized data extraction from this system has supported many research initiatives of the institute. Similarly, SCTIMST has created a fully geo-referenced mapping of its field practice area covering around 35,000 households (a population of 1.32 lakhs) with community participation [ 118 ]. The experiences from such and similar initiatives have given confidence to the state government to undertake more challenging digital health interventions like the e-Health Kerala project. Besides, the various divisions of the medical wing are actively involved in the development of various DHTs in clinical settings.
The Biomedical Technology Wing of the Institute was instrumental in nurturing the Indian medical device industry through know-how development and transfer, providing internationally accredited testing services and offering technology incubation facilities for young entrepreneurs. The BMT wing is actively involved in the development of DHT-enabled medical devices such as para-corporeal left ventricular assist device, centrifugal blood pump with blood flowmeter, deep brain stimulator system for movement disorders, intracranial electrodes, optical peripheral nerve stimulator, 3D printed liver and skin tissue constructs for regenerative applications, PT/INR sensing devices, loop-mediated isothermal amplification-based diagnostic kits, implantable cardioverter defibrillator, programmable hydrocephalus shunt, implantable micro infusion pump with wireless recharging system, and POC kits for sepsis and chlamydia trachomatis. Besides, there are many innovative projects based on smart biomaterials and combinational medical devices under development.
The AMCHSS, the public health division of SCTIMST, was a partner in the customization of the DHIS-2 software for the Indian context, which was piloted in its field practice area in Athiyannur block in Thiruvananthapuram. This led to many field-based research initiatives on digital health, spanning from its use in infectious diseases [ 119 , 120 , 121 , 122 ] to non-communicable diseases [ 123 , 124 , 125 ]. In recent times AMCHSS is moving ahead with infectious disease modeling and the use of data science approaches to large-scale data [ 126 , 127 , 128 , 129 , 130 ]. Lately, the ICMR has entrusted AMCHSS with the analysis of the COVID-19 test data for the entire country. Besides, the AMCHSS is actively involved in the development of various public-health-focused DHTs.
7 Conclusions
Digital health technologies (DHTs) aim to improve the healthcare system across the globe. By providing more accurate diagnoses, enabling more effective treatments and improving patient engagement and compliance, DHTs have the potential to significantly improve patient outcomes. By enabling more efficient and effective delivery of healthcare services and by reducing the need for in-person visits, DHTs can reduce healthcare costs. DHTs can increase access to healthcare through means of remote consultations and support to patients in remote and underserved areas. By compiling patient data from several healthcare providers a more comprehensive digital health record of each patient can be created, providing more accurate and comprehensive patient data to healthcare providers. Thus DHTs can significantly improve healthcare delivery by enabling informed clinical decisions. Further big data can be analyzed by AI-clinical decision support systems to aid the healthcare provider.
Digital health is a rapidly developing field and DHTs are providing new opportunities for innovation and growth, and thus are transforming the medical, pharma, biotech and allied fields. In the medical devices sector, innovations in the field of smart materials, wearable devices, and AI/ML-based systems are rapidly being introduced for clinical use. In the pharma sector, the use of digital technologies has widespread use through various stages of drug development viz. drug design, preclinical validation, and clinical trial. In the biotech and bioengineering sector, digital technologies are aiding in the development of precision and personalized medicinal products. This means there is a growing opportunity for start-ups and established companies to develop new and innovative DHTs. However, a word of caution is necessary to beware of the risks associated with DHTs including ethical and technical concerns.
Digital Health Market Size, Share & Trends Report, 2030. https://www.grandviewresearch.com/industry-analysis/digital-health-market
Digital Health—India. https://www.statista.com/outlook/dmo/digital-health/india
Smartphone-based patient monitoring global market report 2022. https://www.businesswire.com/news/home/20230104005482/en/Smartphone-Based-Patient-Monitoring-Global-Market-Report-2022-Featuring-Leading-Players---Apple-Boston-Scientific-Cerner-Medtronic-and-Phillips-Healthcare---ResearchAndMarkets.com
Dunn J, Runge R, Snyder M (2018) Wearables and the medical revolution. Pers Med 15:429–448. https://doi.org/10.2217/pme-2018-0044
Article Google Scholar
Venkatesan M, Mohan H, Ryan JR et al (2021) Virtual and augmented reality for biomedical applications. Cell Rep Med 2:100348. https://doi.org/10.1016/j.xcrm.2021.100348
Vinolo Gil MJ, Gonzalez-Medina G, Lucena-Anton D et al (2021) Augmented reality in physical therapy: systematic review and meta-analysis. JMIR Serious Games 9:e30985. https://doi.org/10.2196/30985
Chen M, Decary M (2020) Artificial intelligence in healthcare: an essential guide for health leaders. Healthc Manag Forum 33:10–18. https://doi.org/10.1177/0840470419873123
Li L, Lou Z, Chen D et al (2018) Recent advances in flexible/stretchable supercapacitors for wearable electronics. Small 14:1702829. https://doi.org/10.1002/smll.201702829
Choi S, Lee H, Ghaffari R et al (2016) Recent advances in flexible and stretchable bio-electronic devices integrated with nanomaterials. Adv Mater 28:4203–4218. https://doi.org/10.1002/adma.201504150
Gous N, Boeras DI, Cheng B et al (2018) The impact of digital technologies on point-of-care diagnostics in resource-limited settings. Expert Rev Mol Diagn 18:385–397. https://doi.org/10.1080/14737159.2018.1460205
Salem M, Elkaseer A, El-Maddah IAM et al (2022) Non-invasive data acquisition and iot solution for human vital signs monitoring: applications. Limit Future Prospects Sens 22:6625. https://doi.org/10.3390/s22176625
Belushkin A, Yesilkoy F, Altug H (2018) Nanoparticle-enhanced plasmonic biosensor for digital biomarker detection in a microarray. ACS Nano 12:4453–4461. https://doi.org/10.1021/acsnano.8b00519
Kar A, Ahamad N, Dewani M et al (2022) Wearable and implantable devices for drug delivery: applications and challenges. Biomaterials 283:121435. https://doi.org/10.1016/j.biomaterials.2022.121435
Long Y, Li J, Yang F et al (2021) Wearable and implantable electroceuticals for therapeutic electrostimulations. Adv Sci 8:2004023. https://doi.org/10.1002/advs.202004023
Pilotto A, Rizzetti MC, Lombardi A et al (2021) Cerebellar rTMS in PSP: a double-blind sham-controlled study using mobile health technology. Cerebellum 20:662–666. https://doi.org/10.1007/s12311-021-01239-6
Farahani M, Shafiee A (2021) Wound healing: from passive to smart dressings. Adv Healthc Mater 10:2100477. https://doi.org/10.1002/adhm.202100477
Gore JC (2020) Artificial intelligence in medical imaging. Magn Reson Imaging 68:A1–A4. https://doi.org/10.1016/j.mri.2019.12.006
Pugliese L, Marconi S, Negrello E et al (2018) The clinical use of 3D printing in surgery. Update Surg 70:381–388. https://doi.org/10.1007/s13304-018-0586-5
Sun L, Wong Y (2019) Personalized three-dimensional printed models in congenital heart disease. J Clin Med 8:522. https://doi.org/10.3390/jcm8040522
Sirajuddin A, Mirmomen SM, Kligerman SJ et al (2021) Ischemic heart disease: noninvasive imaging techniques and findings. Radiographics 41:E990–E1021. https://doi.org/10.1148/rg.2021200125
Yang D, Martinez C, Visuña L et al (2021) Detection and analysis of COVID-19 in medical images using deep learning techniques. Sci Rep 11:19638. https://doi.org/10.1038/s41598-021-99015-3
Shan R, Sarkar S, Martin SS (2019) Digital health technology and mobile devices for the management of diabetes mellitus: state of the art. Diabetologia 62:877–887. https://doi.org/10.1007/s00125-019-4864-7
Ong DSY, Poljak M (2020) Smartphones as mobile microbiological laboratories. Clin Microbiol Infect 26:421–424. https://doi.org/10.1016/j.cmi.2019.09.026
Coons SJ, Eremenco S, Lundy JJ et al (2015) Capturing patient-reported outcome (PRO) data electronically: the past, present, and promise of epro measurement in clinical trials. Patient Cent Outcomes Res 8:301–309. https://doi.org/10.1007/s40271-014-0090-z
Dinh-Le C, Chuang R, Chokshi S, Mann D (2019) Wearable health technology and electronic health record integration: scoping review and future directions. JMIR MHealth UHealth 7:e12861. https://doi.org/10.2196/12861
Shen Y-T, Chen L, Yue W-W, Xu H-X (2021) Digital technology-based telemedicine for the COVID-19 pandemic. Front Med 8:646506. https://doi.org/10.3389/fmed.2021.646506
Guo C, Ashrafian H, Ghafur S et al (2020) Challenges for the evaluation of digital health solutions—a call for innovative evidence generation approaches. NPJ Digit Med 3:110. https://doi.org/10.1038/s41746-020-00314-2
Kim J, Campbell AS, de Ávila BE-F, Wang J (2019) Wearable biosensors for healthcare monitoring. Nat Biotechnol 37:389–406. https://doi.org/10.1038/s41587-019-0045-y
Koydemir HC, Ozcan A (2018) Wearable and implantable sensors for biomedical applications. Annu Rev Anal Chem 11:127–146. https://doi.org/10.1146/annurev-anchem-061417-125956
Wang C, Xia K, Wang H et al (2019) Advanced carbon for flexible and wearable electronics. Adv Mater 31:1801072. https://doi.org/10.1002/adma.201801072
Correia DM, Fernandes LC, Fernandes MM et al (2021) Ionic liquid-based materials for biomedical applications. Nanomaterials 11:2401. https://doi.org/10.3390/nano11092401
Choi DY, Kim MH, Oh YS et al (2017) Highly stretchable, hysteresis-free ionic liquid-based strain sensor for precise human motion monitoring. ACS Appl Mater Interfaces 9:1770–1780. https://doi.org/10.1021/acsami.6b12415
Yamada S, Toshiyoshi H (2020) Temperature sensor with a water-dissolvable ionic gel for ionic skin. ACS Appl Mater Interfaces 12:36449–36457. https://doi.org/10.1021/acsami.0c10229
Zhang H, Lowe A, Kalra A, Yu Y (2021) A flexible strain sensor based on embedded ionic liquid. Sensors 21:5760. https://doi.org/10.3390/s21175760
Yu Z, Wu P (2021) Water-resistant ionogel electrode with tailorable mechanical properties for aquatic ambulatory physiological signal monitoring. Adv Funct Mater 31:2107226. https://doi.org/10.1002/adfm.202107226
Article MathSciNet Google Scholar
Esteves C, Palma SICJ, Costa HMA et al (2022) Tackling humidity with designer ionic liquid-based gas sensing soft materials. Adv Mater 34:2107205. https://doi.org/10.1002/adma.202107205
Curto VF, Fay C, Coyle S et al (2012) Real-time sweat pH monitoring based on a wearable chemical barcode micro-fluidic platform incorporating ionic liquids. Sens Actuators B Chem 171–172:1327–1334. https://doi.org/10.1016/j.snb.2012.06.048
Zandu SK, Chopra H, Singh I (2020) Ionic liquids for therapeutic and drug delivery applications. Curr Drug Res Rev 12:26–41. https://doi.org/10.2174/2589977511666191125103338
Jian M, Wang C, Wang Q et al (2017) Advanced carbon materials for flexible and wearable sensors. Sci China Mater 60:1026–1062. https://doi.org/10.1007/s40843-017-9077-x
Castro KPR, Colombo RNP, Iost RM et al (2023) Low-dimensionality carbon-based biosensors: the new era of emerging technologies in bioanalytical chemistry. Anal Bioanal Chem. https://doi.org/10.1007/s00216-023-04578-x
Das S, Pal M (2020) Review—non-invasive monitoring of human health by exhaled breath analysis: a comprehensive review. J Electrochem Soc 167:037562. https://doi.org/10.1149/1945-7111/ab67a6
Pang J, Bachmatiuk A, Yang F et al (2021) Applications of carbon nanotubes in the internet of things era. Nano-Micro Lett 13:191. https://doi.org/10.1007/s40820-021-00721-4
Yi J, Xianyu Y (2022) Gold nanomaterials-implemented wearable sensors for healthcare applications. Adv Funct Mater 32:2113012. https://doi.org/10.1002/adfm.202113012
Ali I, Chen L, Huang Y et al (2018) Humidity-responsive gold aerogel for real-time monitoring of human breath. Langmuir 34:4908–4913. https://doi.org/10.1021/acs.langmuir.8b00472
Haine AT, Niidome T (2017) Gold nanorods as nanodevices for bioimaging, photothermal therapeutics, and drug delivery. Chem Pharm Bull (Tokyo) 65:625–628. https://doi.org/10.1248/cpb.c17-00102
Jin H, Jin Q, Jian J (2018) Smart materials for wearable healthcare devices. In: Ortiz JH (ed) Wearable technologies. InTech
Google Scholar
Choo-Smith L-P, Edwards HGM, Endtz HP et al (2002) Medical applications of Raman spectroscopy: from proof of principle to clinical implementation. Biopolymers 67:1–9. https://doi.org/10.1002/bip.10064
Kothari R, Jones V, Mena D et al (2021) Raman spectroscopy and artificial intelligence to predict the Bayesian probability of breast cancer. Sci Rep 11:6482. https://doi.org/10.1038/s41598-021-85758-6
Deore AB, Dhumane JR, Wagh R, Sonawane R (2019) The stages of drug discovery and development process. Asian J Pharm Res Dev 7:62–67. https://doi.org/10.22270/ajprd.v7i6.616
Hughes J, Rees S, Kalindjian S, Philpott K (2011) Principles of early drug discovery: principles of early drug discovery. Br J Pharmacol 162:1239–1249. https://doi.org/10.1111/j.1476-5381.2010.01127.x
Showell GA, Mills JS (2003) Chemistry challenges in lead optimization: silicon isosteres in drug discovery. Drug Discov Today 8:551–556. https://doi.org/10.1016/S1359-6446(03)02726-0
Vemula D, Jayasurya P, Sushmitha V et al (2023) CADD, AI and ML in drug discovery: a comprehensive review. Eur J Pharm Sci 181:106324. https://doi.org/10.1016/j.ejps.2022.106324
Kist R, Timmers LFSM, Caceres RA (2018) Searching for potential mTOR inhibitors: ligand-based drug design, docking and molecular dynamics studies of rapamycin binding site. J Mol Graph Model 80:251–263. https://doi.org/10.1016/j.jmgm.2017.12.015
Aparoy P, Kumar Reddy K, Reddanna P (2012) Structure and ligand based drug design strategies in the development of novel 5- LOX inhibitors. Curr Med Chem 19:3763–3778. https://doi.org/10.2174/092986712801661112
Kanakaveti V, Shanmugam A, Ramakrishnan C et al (2020) Computational approaches for identifying potential inhibitors on targeting protein interactions in drug discovery. In: Advances in protein chemistry and structural biology. Elsevier, pp 25–47
Chikhale RV, Gupta VK, Eldesoky GE et al (2021) Identification of potential anti-TMPRSS2 natural products through homology modelling, virtual screening and molecular dynamics simulation studies. J Biomol Struct Dyn 39:6660–6675. https://doi.org/10.1080/07391102.2020.1798813
Li K, Du Y, Li L, Wei D-Q (2019) Bioinformatics approaches for anti-cancer drug discovery. Curr Drug Targets 21:3–17. https://doi.org/10.2174/1389450120666190923162203
Yu T, Cheng L, Yan X et al (2020) Systems biology approaches based discovery of a small molecule inhibitor targeting both c-Met/PARP-1 and inducing cell death in breast cancer. J Cancer 11:2656–2666. https://doi.org/10.7150/jca.40758
Aldewachi H, Al-Zidan RN, Conner MT, Salman MM (2021) High-throughput screening platforms in the discovery of novel drugs for neurodegenerative diseases. Bioengineering 8:30. https://doi.org/10.3390/bioengineering8020030
Ferdowsian HR, Beck N (2011) Ethical and scientific considerations regarding animal testing and research. PLoS ONE 6:e24059. https://doi.org/10.1371/journal.pone.0024059
Achary PGR (2020) Applications of quantitative structure-activity relationships (QSAR) based virtual screening in drug design: a review. Mini-Rev Med Chem 20:1375–1388. https://doi.org/10.2174/1389557520666200429102334
Staszak M, Staszak K, Wieszczycka K et al (2022) Machine learning in drug design: Use of artificial intelligence to explore the chemical structure–biological activity relationship. WIREs Comput Mol Sci. https://doi.org/10.1002/wcms.1568
Raies AB, Bajic VB (2016) In silico toxicology: computational methods for the prediction of chemical toxicity: computational methods for the prediction of chemical toxicity. Wiley Interdiscip Rev Comput Mol Sci 6:147–172. https://doi.org/10.1002/wcms.1240
Workman P (2003) How much gets there and what does it do?: The need for better pharmacokinetic and pharmacodynamic endpoints in contemporary drug discovery and development. Curr Pharm Des 9:891–902. https://doi.org/10.2174/1381612033455279
Bouzom F, Ball K, Perdaems N, Walther B (2012) Physiologically based pharmacokinetic (PBPK) modelling tools: how to fit with our needs?: PBPK MODELLING TOOLS. Biopharm Drug Dispos 33:55–71. https://doi.org/10.1002/bdd.1767
Mahan VL (2014) Clinical trial phases. Int J Clin Med 05:1374–1383. https://doi.org/10.4236/ijcm.2014.521175
Gold M, Amatniek J, Carrillo MC et al (2018) Digital technologies as biomarkers, clinical outcomes assessment, and recruitment tools in Alzheimer’s disease clinical trials. Alzheimers Dement Transl Res Clin Interv 4:234–242. https://doi.org/10.1016/j.trci.2018.04.003
Bennett AV, Jensen RE, Basch E (2012) Electronic patient-reported outcome systems in oncology clinical practice. CA Cancer J Clin 62:336–347. https://doi.org/10.3322/caac.21150
Gong K, Yan Y-L, Li Y et al (2020) Mobile health applications for the management of primary hypertension: a multicenter, randomized, controlled trial. Medicine (Baltimore) 99:e19715. https://doi.org/10.1097/MD.0000000000019715
Gordon S, Crager J, Howry C et al (2022) Best practice recommendations: user acceptance testing for systems designed to collect clinical outcome assessment data electronically. Ther Innov Regul Sci 56:442–453. https://doi.org/10.1007/s43441-021-00363-z
Galsky MD, Shahin M, Jia R et al (2017) Telemedicine-enabled clinical trial of metformin in patients with prostate cancer. JCO Clin Cancer Inform. https://doi.org/10.1200/CCI.17.00044
Beg S, Handa M, Shukla R et al (2022) Wearable smart devices in cancer diagnosis and remote clinical trial monitoring: transforming the healthcare applications. Drug Discov Today 27:103314. https://doi.org/10.1016/j.drudis.2022.06.014
Patel VN, Kaelber DC (2014) Using aggregated, de-identified electronic health record data for multivariate pharmacosurveillance: a case study of azathioprine. J Biomed Inform 52:36–42. https://doi.org/10.1016/j.jbi.2013.10.009
Needamangalam Balaji J, Prakash S, Park Y et al (2022) A scoping review on accentuating the pragmatism in the implication of mobile health (mHealth) technology for tuberculosis management in India. J Pers Med 12:1599. https://doi.org/10.3390/jpm12101599
Holmén C, Piehl F, Hillert J et al (2011) A Swedish national post-marketing surveillance study of natalizumab treatment in multiple sclerosis. Mult Scler J 17:708–719. https://doi.org/10.1177/1352458510394701
Antonijević Z, Beckman RA (2019) Platform trial designs in drug development: umbrella trials and basket trials. CRC Press, Boca Raton
Battaglini D, Al-Husinat L, Normando AG et al (2022) Personalized medicine using omics approaches in acute respiratory distress syndrome to identify biological phenotypes. Respir Res 23:318. https://doi.org/10.1186/s12931-022-02233-0
Poirion OB, Jing Z, Chaudhary K et al (2021) DeepProg: an ensemble of deep-learning and machine-learning models for prognosis prediction using multi-omics data. Genome Med 13:112. https://doi.org/10.1186/s13073-021-00930-x
Tran KA, Kondrashova O, Bradley A et al (2021) Deep learning in cancer diagnosis, prognosis and treatment selection. Genome Med 13:152. https://doi.org/10.1186/s13073-021-00968-x
Turek C, Wróbel S, Piwowar M (2020) OmicsON—integration of omics data with molecular networks and statistical procedures. PLoS ONE 15:e0235398. https://doi.org/10.1371/journal.pone.0235398
Galeone C, Scelfo C, Bertolini F et al (2018) Precision medicine in targeted therapies for severe asthma: is there any place for “omics” technology? BioMed Res Int 2018:1–15. https://doi.org/10.1155/2018/4617565
Big data analytics in healthcare market. https://www.factmr.com/report/369/big-data-analytics-healthcare-market
Hemingway H, Asselbergs FW, Danesh J et al (2018) Big data from electronic health records for early and late translational cardiovascular research: challenges and potential. Eur Heart J 39:1481–1495. https://doi.org/10.1093/eurheartj/ehx487
Wang L, Alexander CA (2020) Big data analytics in medical engineering and healthcare: methods, advances and challenges. J Med Eng Technol 44:267–283. https://doi.org/10.1080/03091902.2020.1769758
Koppad S, B A, Gkoutos GV, Acharjee A, (2021) Cloud computing enabled big multi-omics data analytics. Bioinforma Biol Insights 15:1177932221. https://doi.org/10.1177/11779322211035921
Morris MA, Saboury B, Burkett B et al (2018) Reinventing radiology: big data and the future of medical imaging. J Thorac Imaging 33:4–16. https://doi.org/10.1097/RTI.0000000000000311
Ngiam KY, Khor IW (2019) Big data and machine learning algorithms for health-care delivery. Lancet Oncol 20:e262–e273. https://doi.org/10.1016/S1470-2045(19)30149-4
Ho D, Quake SR, McCabe ERB et al (2020) Enabling technologies for personalized and precision medicine. Trends Biotechnol 38:497–518. https://doi.org/10.1016/j.tibtech.2019.12.021
Sadler D, Okwuosa T, Teske AJ et al (2022) Cardio oncology: Digital innovations, precision medicine and health equity. Front Cardiovasc Med 9:951551. https://doi.org/10.3389/fcvm.2022.951551
Vajawat B, Varshney P, Banerjee D (2021) Digital gaming interventions in psychiatry: evidence. Appl Chall Psych Res 295:113585. https://doi.org/10.1016/j.psychres.2020.113585
Raijada D, Wac K, Greisen E et al (2021) Integration of personalized drug delivery systems into digital health. Adv Drug Deliv Rev 176:113857. https://doi.org/10.1016/j.addr.2021.113857
Montanhesi PK, Coelho G, Curcio SAF, Poffo R (2022) Three-dimensional printing in minimally invasive cardiac surgery: optimizing surgical planning and education with life-like models. Braz J Cardiovasc Surg. https://doi.org/10.21470/1678-9741-2020-0409
Jamróz W, Szafraniec J, Kurek M, Jachowicz R (2018) 3D printing in pharmaceutical and medical applications—recent achievements and challenges. Pharm Res 35:176. https://doi.org/10.1007/s11095-018-2454-x
Bedford J, Farrar J, Ihekweazu C et al (2019) A new twenty-first century science for effective epidemic response. Nature 575:130–136. https://doi.org/10.1038/s41586-019-1717-y
Canfell OJ, Davidson K, Woods L et al (2022) Precision public health for non-communicable diseases: an emerging strategic roadmap and multinational use cases. Front Public Health 10:256
Cubillos-Ruiz A, Guo T, Sokolovska A et al (2021) Engineering living therapeutics with synthetic biology. Nat Rev Drug Discov 20:941–960. https://doi.org/10.1038/s41573-021-00285-3
Davies JA (2016) Synthetic biology: rational pathway design for regenerative medicine. Gerontology 62:564–570. https://doi.org/10.1159/000440721
Sridhar S, Ajo-Franklin CM, Masiello CA (2022) A framework for the systematic selection of biosensor chassis for environmental synthetic biology. ACS Synth Biol 11:2909–2916. https://doi.org/10.1021/acssynbio.2c00079
Jain KK (2013) Synthetic biology and personalized medicine. Med Princ Pract 22:209–219. https://doi.org/10.1159/000341794
McNerney MP, Doiron KE, Ng TL et al (2021) Theranostic cells: emerging clinical applications of synthetic biology. Nat Rev Genet 22:730–746. https://doi.org/10.1038/s41576-021-00383-3
Zupanic A, Bernstein HC, Heiland I (2020) Systems biology: current status and challenges. Cell Mol Life Sci 77:379–380. https://doi.org/10.1007/s00018-019-03410-z
Lopatkin AJ, Collins JJ (2020) Predictive biology: modelling, understanding and harnessing microbial complexity. Nat Rev Microbiol 18:507–520. https://doi.org/10.1038/s41579-020-0372-5
Irmisch A, Bonilla X, Chevrier S et al (2021) The Tumor Profiler Study: integrated, multi-omic, functional tumor profiling for clinical decision support. Cancer Cell 39:288–293. https://doi.org/10.1016/j.ccell.2021.01.004
McEwen SC, Merrill DA, Bramen J et al (2021) A systems-biology clinical trial of a personalized multimodal lifestyle intervention for early Alzheimer’s disease. Alzheimers Dement Transl Res Clin Interv. https://doi.org/10.1002/trc2.12191
Wolstencroft K, Owen S, Krebs O et al (2015) SEEK: a systems biology data and model management platform. BMC Syst Biol 9:33. https://doi.org/10.1186/s12918-015-0174-y
Kobeissy FH, Guingab-Cagmat JD, Razafsha M et al (2011) Leveraging biomarker platforms and systems biology for rehabilomics and biologics effectiveness research. PM&R 3:S139–S147. https://doi.org/10.1016/j.pmrj.2011.02.012
Brown S-A (2015) Building SuperModels: emerging patient avatars for use in precision and systems medicine. Front Physiol. https://doi.org/10.3389/fphys.2015.00318
Cummins N, Schuller BW (2020) Five crucial challenges in digital health. Front Digit Health 2:536203. https://doi.org/10.3389/fdgth.2020.536203
Board of Governors in Supersession of the Medical Council of India (2020) Telemedicine practice guidelines-enabling registered medical practitioners to provide healthcare using telemedicine
Latifi R, Doarn CR (2020) Perspective on COVID-19: finally, telemedicine at center stage. Telemed E-Health 26:1106–1109. https://doi.org/10.1089/tmj.2020.0132
Gudi N, Lakiang T, Pattanshetty S et al (2021) Challenges and prospects in india’s digital health journey. Indian J Public Health 65:209. https://doi.org/10.4103/ijph.IJPH_1446_20
Dash S, Aarthy R, Mohan V (2021) Telemedicine during COVID-19 in India—a new policy and its challenges. J Public Health Policy 42:501–509. https://doi.org/10.1057/s41271-021-00287-w
Srivastava SK (2016) Adoption of electronic health records: a roadmap for India. Healthc Inform Res 22:261. https://doi.org/10.4258/hir.2016.22.4.261
Madanian S, Parry DT, Airehrour D, Cherrington M (2019) mHealth and big-data integration: promises for healthcare system in India. BMJ Health Care Inform 26:e100071. https://doi.org/10.1136/bmjhci-2019-100071
Al Dahdah M, Mishra RK (2023) Digital health for all: the turn to digitized healthcare in India. Soc Sci Med 319:114968. https://doi.org/10.1016/j.socscimed.2022.114968
Katyayan A, Katyayan A, Mishra A (2022) Enhancing India’s health care during COVID era: role of artificial intelligence and algorithms. Indian J Otolaryngol Head Neck Surg 74:2712–2713. https://doi.org/10.1007/s12070-020-02101-7
National Digital Health Mission. https://www.sanskritiias.com/current-affairs/national-digital-health-mission
Soman B (2014) Participatory GIS in action, a public health initiative from Kerala, India. ISPRS Int Arch Photogramm Remote Sens Spat Inf Sci XL–8:233–237. https://doi.org/10.5194/isprsarchives-XL-8-233-2014
Babu AN, Niehaus E, Shah S et al (2019) Smartphone geospatial apps for dengue control, prevention, prediction, and education: MOSapp, DISapp, and the mosquito perception index (MPI). Environ Monit Assess 191:393. https://doi.org/10.1007/s10661-019-7425-0
Chaudhary S, Soman B (2022) Spatiotemporal analysis of environmental and physiographic factors related to malaria in Bareilly district, India. Osong Public Health Res Perspect 10:10. https://doi.org/10.24171/j.phrp.2021.0304
Singh G, Mitra A, Soman B (2022) Development and use of a reproducible framework for spatiotemporal climatic risk assessment and its association with decadal trend of dengue in India. Indian J Community Med 47:50. https://doi.org/10.4103/ijcm.ijcm_862_21
Valson JS, Soman B (2017) Spatiotemporal clustering of dengue cases in Thiruvananthapuram district. Kerala’ Indian J Public Health 61:74
Sarma PS, Sadanandan R, Thulaseedharan JV et al (2019) Prevalence of risk factors of non-communicable diseases in Kerala, India: results of a cross-sectional study. BMJ Open. https://doi.org/10.1136/bmjopen-2018-027880
Ulahannan SK, Wilson A, Chhetri D et al (2022) Alarming level of severe acute malnutrition in Indian districts. BMJ Glob Health 7:e007798. https://doi.org/10.1136/bmjgh-2021-007798
Valson JS, Kutty VR, Soman B, Jissa VT (2019) Spatial clusters of diabetes and physical inactivity: do neighborhood characteristics in high and low clusters differ? Asia Pac J Public Health. https://doi.org/10.1177/1010539519879322
Mitra A, Soman B, Singh G (2021) An interactive dashboard for real-time analytics and monitoring of COVID-19 outbreak in india: a proof of Concept. arXiv:210809937Cs
Mitra A, Soman B, Gaitonde R et al (2023) Data science approaches to public health: case studies using routine health data from India
Singh G, Patrikar S, Sarma PS, Soman B (2020) Time-dependent dynamic transmission potential and instantaneous reproduction number of COVID-19 pandemic in India. medRxiv. https://doi.org/10.1101/2020.07.15.20154971
Singh G, Srinivas G, Jyothi EK et al (2020) Containing the first outbreak of COVID-19 in a healthcare setting in India: the sree chitra experience. Indian J Public Health 64:240. https://doi.org/10.4103/ijph.IJPH_483_20
Singh G, Soman B (2021) Spatiotemporal epidemiology and forecasting of dengue in the state of Punjab, India: study protocol. Spat Temp Epidemiol 39:100444. https://doi.org/10.1016/j.sste.2021.100444
Download references
Author information
Authors and affiliations.
Sree Chitra Tirunal Institute for Medical Science and Technology, Thiruvananthapuram, 695011, Kerala, India
Naresh Kasoju, N. S. Remya, Renjith Sasi, S. Sujesh, Biju Soman, C. Kesavadas, C. V. Muraleedharan, P. R. Harikrishna Varma & Sanjay Behari
You can also search for this author in PubMed Google Scholar
Contributions
All authors listed have made an equal and substantial contribution to the work.
Corresponding author
Correspondence to Sanjay Behari .
Ethics declarations
Conflict of interest.
The authors have no relevant financial or non-financial conflicts of interest to disclose.
Rights and permissions
Reprints and permissions
About this article
Kasoju, N., Remya, N.S., Sasi, R. et al. Digital health: trends, opportunities and challenges in medical devices, pharma and bio-technology. CSIT 11 , 11–30 (2023). https://doi.org/10.1007/s40012-023-00380-3
Download citation
Received : 17 February 2023
Accepted : 27 March 2023
Published : 11 April 2023
Issue Date : April 2023
DOI : https://doi.org/10.1007/s40012-023-00380-3
Share this article
Anyone you share the following link with will be able to read this content:
Sorry, a shareable link is not currently available for this article.
Provided by the Springer Nature SharedIt content-sharing initiative
- Medical devices
- Telemedicine
- Biomaterials
- Drug discovery
- Bioengineering
- Find a journal
- Publish with us
- Track your research
- Open access
- Published: 25 September 2021
Biomedical engineering and ethics: reflections on medical devices and PPE during the first wave of COVID-19
- Alessia Maccaro 1 , 2 na1 ,
- Davide Piaggio ORCID: orcid.org/0000-0001-5408-9360 1 na1 ,
- Concetta Anna Dodaro 3 &
- Leandro Pecchia 1 , 4 , 5
BMC Medical Ethics volume 22 , Article number: 130 ( 2021 ) Cite this article
15k Accesses
7 Citations
2 Altmetric
Metrics details
In March 2019, the World Health Organization (WHO) declared that humanity was entering a global pandemic phase. This unforeseen situation caught everyone unprepared and had a major impact on several professional categories that found themselves facing important ethical dilemmas. The article revolves around the category of biomedical and clinical engineers, which were among those most involved in dealing with and finding solutions to the pandemic. In hindsight, the major issues brought to the attention of biomedical engineers have raised important ethical implications, such as the allocation of resources, the responsibilities of science and the inadequacy and non-universality of the norms and regulations on biomedical devices and personal protective equipment. These issues, analyzed one year after the first wave of the pandemic, come together in the appeal for responsibility for thought, action and, sometimes, even silence. This highlights the importance of interdisciplinarity and the definitive collapse of the Cartesian fragmentation of knowledge, calling for the creation of more fora, where this kind of discussions can be promoted.
Peer Review reports
Since early 2020, the current SARS-CoV-2 pandemic has challenged all the fields of knowledge, increasing the need for their interconnection. Medicine, science, politics, and more specialized sectors such as biomedical engineering (BME), faced crucial ethical issues, which can no longer be underestimated.
Biomedical engineers design medical devices, raising many ethical dilemmas in ordinary times, which become compelling during such a crisis. The authors of this manuscript had the privilege of different points of view thanks to the President of the European Society of BME (i.e., EAMBES) and Secretary General of the global society of BME and medical physics (IUPESM).
Three pivotal themes emerged in the global BME community:
The dilemma of identifying criteria for the allocation of medical devices
Responsibilities of science and technology
Inadequacy of regulations and norms, which lack universality
This manuscript does not follow the traditional structure of scientific papers (e.g., methods, results etc.), rather it is a critical analysis, revolving around the 3 above-mentioned pillars. The first pillar focuses on the surfacing of ethical dilemmas in times of pandemic (e.g., scarce resource allocation), delving into examples from Italy, where the new decision-making criteria often clashed against the existing constitutional and moral principles during the first wave.
The second pillar retrospectively reports how the medical device sector was affected by the current pandemic, touching on hazardous amatorial attempts of the general public to face the scarcity of resources and the urgent needs, and on the crisis-related challenges that surfaced for manufacturers, exacerbated by the lack of dialogue with decision-makers. In the same pillar, the theoretical debate among science and politics is addressed, referring the case of the “intended use” of a medical device, from two different philosophical perspectives, i.e., substantialism and utilitarianism. The former underlines the fundamentality of substances as ontological categories [ 1 ], suggesting that the intended use of a medical device should always be respected. The latter stresses the importance of maximizing the overall good, authorizing different uses, depending on the relativistic utility or, in exceptional times of crisis, on the emergency.
This invites the reader to a subsequent reflection on the inadequacy of the existing regulations on medical devices, in the third pillar. In respect to this, the manuscript proposes to offer a hermeneutic perspective close to the situational ethics that authorizes negotiations and mediations between the generality of principles and norms, and the specificity of the context.
Overall, the ethical considerations made in this manuscript, should be considered a valuable lesson for the future of crisis management. If and only if ethics and bioethics will be considered as effective support for science and scientists (doctors, biomedical engineers, etc.), the Cartesian separation of knowledge [ 2 ] could be overcome, establishing an interdisciplinary dialogue that involves peoples and emphasizes the public relevance of such issues.
In this way, such dilemmas could be anticipated by establishing a framework that could provide guidance and appropriate methodology to address arising urgent issues, without having to resort to specialists for questions that concern everyone and need a multidisciplinary approach.
Allocation of medical devices: clinical and ethical principles
Due to limited resources, decision-makers have had to compromise among all the potential useful interventions. Also in the past 10 years, the most public National health systems were massively privatized, resulting in a significant reduction of prevention services and a great reduction of Intensive Care Unit (ICU) beds. The rapid spread of the SARS-CoV-2 resulted in an unprecedented need of sub-intensive and ICU beds, which overcame the capacity of the most advanced national healthcare services. Within a few weeks, the available resources (i.e., medical devices, doctors, nurses) proved to be insufficient to cover the care needs of the multitude of COVID-19 patients, beyond the ordinary needs of other patients. Consequently, doctors and healthcare structures ended up pondering and making difficult ethical choices in a short time and identifying priority principles that could guide them.
National [ 3 , 4 , 5 , 6 ] and international [ 7 , 8 , 9 ] ethics committees, scientific societies [ 10 , 11 ], and experts [ 12 , 13 , 14 , 15 ] soon expressed their opinion on the matter [ 16 ], identifying requirements that respect human dignity and fundamental ethical principles, enshrined in the Charters of both National and International Rights [ 17 ].
The case of Italy, the first country significantly affected outside China, is emblematic. According to the Italian Constitution, health is a "fundamental right of the individual" and a "collective interest" (article 32). In addition, article 2 recognizes the personalist principle and the duty of solidarity, and article 3 establishes the principle of equality. Accordingly, the law founding the Italian National Health Services (NHS) (n. 883/1978) prescribes that care must be ensured according to the principles of universality, equality and fairness.
Leveraging on these fundamental principles, the Italian National Bioethics Committee [ 18 ] remarked the need to allocate medical devices and other resources based only on a clinical criterion, and without considering criteria such as age, gender, social attributes, ethnicity, disability and costs, in compliance with the principles of "justice, equity, solidarity". The envisaged method was that of "triage in a pandemic emergency", based on what the World Health Organization defines as "preparedness" (WHO) as a premise, and on two key concepts, i.e., "clinical appropriateness" and "actuality", identified by the healthcare professional on the basis of clinical criteria [ 19 ].
This point of view, which values the person and opposes attempts of objectification in a series of “pre-established criteria” (except for the point of view of the clinician), is well expressed by J. Habermas. In an interview with the newspaper Le Monde, Habermas underlined the inadequacy, in moral terms, of an “objective quantification” of patients and insisted on the essential issue of the “recognition” of the individual: “when addressing a second person (you, you), the other person's self-determination must either be respected or denied, that is, either accepted or ignored” [ 20 ].
On the other hand, the Italian society of anesthesiology (SIAARTI) introduced the identification of an age limit for accessing intensive care in case of necessity [ 21 ]. SIAARTI’s document explained that COVID-19 created a scenario, in which criteria for accessing ICU may be needed beyond the clinical appropriateness and proportionality of care, but also in distributive justice and the appropriate allocation of limited healthcare resources, which means privileging those with the "greatest life expectancy" [ 22 ]. Even if the choice of whom to admit to treatment is a terrible reality, it must be discussed by ethicists and bioethicists to further identify solutions that support medical doctors in taking these decisions. “This is not to deny their clinical authority and responsibility, but rather to urge a commitment to give such question public relevance" so that in the future the "public health perspective" [ 23 ] to follow is clear from the beginning.
Responsible thinking, responsible actions, responsible silence
COVID-19 created a global lack of essential medical devices (e.g., pulmonary ventilators) and personal protective equipment (PPE, such as masks, respirators) [ 24 ]. This led to an unprecedented amount of do-it-yourself (DIY) solutions, which were fomented on media worldwide. Consequently, ordinary individuals started producing PPE at home with 3D printers and everyday materials, and manufacturers converted their production facilities to develop medical devices and PPE.
Unfortunately, although very admirable, this approach is not feasible in critical sectors such as medical devices or PPE, which require postgraduate education, years of experience and deep knowledge of relevant international standards and norms, in order to ensure appropriate levels of safety, efficacy and resilience. Thus, only 7 manufacturers in the world are producing pulmonary ventilators. In fact, during a pandemic, we do not only need to ensure the usual standard of quality, but we should also consider making those devices more resilient, because, if hospitals fail, we will need to safely operationalize these devices in field hospitals, tent-like structures, and any other relevant setting. Therefore, experience, safe-by-design approaches, and the knowledge of additional standards (e.g., military standards) become relevant too.
Minimal scientific evidence exists on how harmful this DIY wave has been, but few facts can be clearly reported referencing major newspapers. In mid-March 2020, manufacturers were called upon to help to tackle medical devices and PPE crisis. Footnote 1 Footnote 2 Many responded, certainly moved by the noblest principle and willingness to help. Unfortunately, learning to manufacture complicated and highly regulated pulmonary ventilators cannot be done in a few weeks. By mid-April 2020, new productions of ventilators were stopped in Spain, Footnote 3 UK suspended orders of BlueSky ventilators Footnote 4 and France followed. Footnote 5 Once again, a virtuous example came from Italy, where the only Italian manufacturer of ventilators (i.e., Siare Engineering International Group l.t.d.) was supported by the Italian Government, which offered 25 highly specialized army engineers, by the former FIAT (now FCA), who supported producing electro-mechanic components, in addition to Ferrari providing electronic components. With this collaborative effort, Siare increased the production of top-of-the-range ventilators from 160 to 500 units per month, respecting the highest quality standards. In conclusion, the rise of useless and potentially harmful DIY approaches to PPE and medical devices could have been easily avoided at the start of the pandemic by decision-makers initially consulting with domain experts, such as biomedical and clinical engineers.
In this regard, the belief that disciplinary competence is to be sectorized and not interconnected, very often, leads to a further separation of knowledge that is detrimental to people. In fact, it could be good practice that politicians had a solid scientific background in order to legislate about scientific matters, above all if they involve public health. On the other hand, scientists should "grovel in the dirt of the city of Romulus" [ 25 ] keeping their related studies as tangible and accessible as possible, and acquire a more solid political culture and a growing awareness of their social role. After all, the relationship between science, policy-making and politics has been controversial since the dawns of civilization: people like Aristarchus of Samos (i.e., one of the fathers of an early Heliocentrism), censored by sectaries such as Cleanthes (i.e., the prince of stoics at that age), or like Socrates, accused, “censored”, and sentenced to death for being “unorthodox” by one of the most open and democratic societies of the times, the Polis of Athens [ 26 ], or like Galileus, who was condemned for radically opposing to the Sacred Scriptures- and Church-approved Geocentric model, are just a few stark examples. Although much progress has been made since then, there is still an ongoing debate among a Weberian distinction between science and politics and a Habermasian and Marcusean dichotomy between the technocratic and decisionist models of scientific advice to politics. Nonetheless, it is evident that we are transitioning towards ever more present democratization of science, and not without associated risks. In fact, how can this be achieved without compromising the epistemic quality of knowledge [ 27 ]?
In particular, the COVID-19-related debate, involving politicians, scientists (especially biomedical engineers) and ethics experts, is based on two distinct currents of thought, referring to two different philosophical matrices, i.e., utilitarianism and substantialism . In fact, some people believe that any kind of emergency-ready response that can make up for the shortage of PPE and medical devices is “better than nothing”, even at the expense of the safety and efficiency normally guaranteed by the standards. This way of thinking is in line with Utilitarianism’s conception of maximizing happiness and overall gains for all the affected individuals. However, the “better than nothing approach” is dubious and is a well-known logical fallacy, that of the relative privation [ 28 ]. This kind of fallacious way of reasoning also justifies the misuse of something that does meet the standards but was intended for completely different purposes. In fact, the intended purpose is key, at least in the world of medical devices, and it is what safeguards the manufacturers in case their products fail if they are used “off label”. In this case, the liability falls with the individual who misused the product in the first place [ 29 ]. This concept is well portrayed by what happened in Harrow, Footnote 6 , Footnote 7 where some nurses, after denouncing their precarious working conditions and the lack of PPE in the fight against COVID-19, had started using bin bags as a replacement of the unavailable PPE. In this case, the beneficial objective was given priority and the collective benefit was maximised. However, also the risk to the wearer had rapidly increased to the extent that it was not possible to predict its consequences (even negative).
Conversely, other people believe that the intended purpose of an object should be respected, in line with Substantialism’s theories, which attribute absolute value to an idea. According to this perspective, for example, a bin bag would be designed, tested and marketed to contain rubbish, not to protect healthcare workers from diseases (in this case the design principles and the tests will be different and stricter). Thus, these people tend towards a minimisation of the risk, but, at the same time, their precautional approach hinders the possible benefit underlying the other “less safe” alternatives. In this regard, it is necessary to recall the philosophy of Hans Jonas who, faced with an indeterminate and potential risk, the consequences of which cannot be estimated, introduced the imperative of responsibility in defence of future generations and based on the precautionary principle. His "heuristics of fear" implies foresight and ability to predict and adequately assess the consequences of collective activities in contemporary societies. Such principle implies to “act so that the effects of your action are compatible with the permanence of genuine life” and, in our present choices, to “include the future wholeness of Man among the objects of your (our) will” [ 30 ].
Consequently, this responsibility goes beyond the personal one of engineers, as it also includes the responsibility they partially assume if and when they do not limit reckless or inadequately considered actions, guided by the above-mentioned utilitarian approach. In fact, the compliance with international standards and the consequent CE marking does not only guarantee the quality, safety, efficiency, and efficacy of a product, but also the protection of manufacturers and users. In fact, as afore-mentioned from a general point of view, those to be blamed for the possible failure of bin bags used as PPE for the prevention of COVID-19 and the consequent infection and potential death of the healthcare workers using them are not the manufacturers of such items, but whomever decided to use this amateur substitution to other certified means of protection, and, to a certain extent, the biomedical engineers who did not respect their duty to identify the limits regarding the unintended uses.
Overall, two theoretical orientations are at the basis of these dichotomous approaches. However, in order to better frame them, it is necessary to analyse them in view of the extraordinary condition of necessity begotten by the pandemic. The dilemma revolves around the "intended use", or rather the purpose for which something (e.g., PPE or medical devices) was originally designed for: on the one hand there are those who assert that, in conditions of necessity, the contingent purpose, i.e., the social functionality that overcomes the intended use, ought to be preferred despite being “off label”. In fact, they firmly believe that it is preferable to maximize the current benefit while assuming an undefined risk. Although it is not easy to relate this trend to a specific current of thought, it certainly shares some points in common with utilitarianism, starting from the Benthamian one [ 31 ], if not with pragmatism (e.g., Dewey [ 32 ]).
On the other hand, the position of those who consider the “intended use” or rather the intrinsic purpose for which the product was manufactured tested and marketed, a priority, would seem evocative of Aristotelian substantialism or eschatology. In this case, the risks are limited by compliance with the law and the relevant standards, which also guarantee the achievement of the benefits. The refusal of this immediately relieves the manufacturer and the regulator from any responsibility related to the misuse of the object, leaving every possible and unforeseeable risk to the individual.
Here lies the crux of the problem. Using what is available and certified, albeit designed for a different “intended use”, seems more “reasonable” than not protecting oneself to everyone. However, we cannot refrain from asking ourselves the following questions: what is the limit within which it is possible to say, “better than nothing”? To what extent can science and policymakers put people's lives at risk in order to have a prompt, but probably unsafe answer in the wake of the "better than nothing" principle?
Regulatory frameworks and standards should be reviewed in this regard.
Beyond the DIY solutions, low-quality outputs have been affecting scientific production too. In fact, the high demand for information caused an acceleration in reporting scientific results, with many journals being overwhelmed with unprecedented numbers of papers, which challenged the capability of editors and reviewers to scrutinise articles.
The unprecedented high number of retracted papers can be a proxy for the high number of low-quality research on COVID-19. For this reason, a rapid search for papers regarding COVID-19 or SARS-CoV-2, and the previous epidemic/pandemics (i.e., SARS, MERS, Swine Flu) as a comparison, was performed both on OvidSP and the Retraction Watch Database. As regards COVID-19 publications, there were 124 retracted papers out of 264,530, i.e., 4.68 per 10,000 papers (compared to 1.16 per 10,000 papers concerning the previous pandemics/epidemics). Although this proxy is to be taken with a grain of salt, it should be a wake-up call for further investigations. Similar levels of confusion could be observed also among scientists and experts invited by media to interpret available scientific evidence and technical guidance.
The above-mentioned issues contributed to beget and feed an infodemic , defined by the United Nation as “an over-abundance of information—some accurate and some not—that makes it hard for people to find trustworthy sources and reliable guidance when they need it” [ 33 ], which is inducing an unprecedented need for responsible silence too.
Inadequacy of regulatory frameworks and norms
The pandemic creates a generalized condition of resource limited settings (RLSs), i.e., environments lacking means, specific knowledge, specialized personnel, medical devices and drugs within inappropriate medical locations. While this condition was already familiar to low- and middle-income countries, COVID-19 has overwhelmingly created RLS conditions in high-income countries, such as Europe, the USA and Japan, for the first time since World War II. This demonstrates how regulatory frameworks for medical devices and PPE are inadequate to RLS conditions. In fact, these regulations usually take into consideration standards that are too stringent and generic, proving impossible to fulfil in RLSs and, in times of the pandemic, difficult to adhere to universally. For example, the numerous tests and verifications required to assess the conformity of market respiratory protective equipment or eye protection equipment for healthcare purposes wasted time. One reason for this is that these standards are influenced by big manufacturers interested in having the largest market share. As a result, commercial standards for PPEs require testing in conditions that are not relevant for hospital workers (e.g., high temperature typical of heavy metals industry). Hence, international standards and norms followed the principle of generalism, losing universality and creating unnecessary burdens for small manufacturers [ 34 ]. In this regard, the WHO has published, for the first time, technical guidance on PPE specifically relevant for healthcare settings [ 35 , 36 ]. Differently to ISO standards for masks and respirators, the WHO guidance focuses on essential parameters, such as filtering capability, fit and breathability for masks.
Recalling the two aforementioned ethical perspectives, considering existing medical device regulation too generic to be universal, does not mean adhering to the utilitarian-pragmatic current tout court. There may be a contextualised response, regulated on the basis of tests, complying with flexible standards, or rather standards that are purposely designed to take into account different niche conditions. However, the use of any object must be certified and not random, and subject to tests relating to its specific intended use. Only in this way, people and their rights can be safeguarded, and science can prove to think deeply, act consciously and remain silent, when appropriate.
Contextualism is the basis of situational ethics [ 37 ], which seems to be the most adequate response to the specific needs of everyone and be able to face emergencies. In fact, it starts from the particular situation and tries to find universalizable answers, applying a heuristic and inductive method, progressive negotiations and interdisciplinary exchanges.
Conclusions
One year after the start of the pandemic, the need for ethic guidance is still tangible in everyday circumstances and essential during crisis or in RLSs. Respecting fundamental ethical principles while negotiating among different criteria (hospitalization demands vs available ICUs, generalism vs particularism, action vs responsible-action) requires clear guidance, deep knowledge, and peer-to-peer discussion among experts of different disciplines. The need for extreme specialization should never result in the fragmentation of knowledge.
Exactly a century, i.e., the Short Twentieth Century , separates COVID-19 from the last pandemic, the so-called "Spanish Flu", which flagellated Europe in 1918–1919. According to Hobsbawm, “ no period in history has been more penetrated by and more dependent on the natural sciences ” and “ yet no period, since Galileo's recantation, has been less at ease with it ”. This chasm between scientists and the general public is still open and, in some cases, fomented by populisms, which leverage on people's fears evoking war atmospheres, which have nothing to do with the catastrophic failure of many national healthcare systems’ response to this crisis. After a century, the dependence of medicine on biomedical science and engineering is evident, while their contribution to the definition of effective policies and norms is still negligible. Finally, the Cartesian fragmentation of knowledge, or rather “thinking in silos”, has persisted across the last century, calling for the creation of more fora where multidisciplinary discussions can be promoted. Three main needs emerged clearly: the need for responsible thinking, the need for responsible action and the need for responsible silence, when required and appropriate [ 38 ].
Availability of data and materials
Not applicable.
https://www.ft.com/content/491a4af4-66e7-11ea-a3c9-1fe6fedcca75 .
https://www.nytimes.com/2020/03/20/us/politics/trump-coronavirus-supplies.html .
https://www.lavanguardia.com/vida/20200411/48413026980/seat-detiene-produccion-respidradores-emergencia-descongestion-uci.html .
https://uk-mobile-reuters-com.cdn.ampproject.org/c/s/uk.mobile.reuters.com/article/amp/idUKKCN21U0UI .
https://www.franceinter.fr/coronavirus-8-500-respirateurs-produits-pour-rien .
https://www.bbc.co.uk/news/health-52145140 .
https://www.telegraph.co.uk/news/2020/04/08/exclusive-three-nurses-forced-wear-bin-bags-ppe-shortage-test/ .
Abbreviations
World Health Organization
Biomedical Engineering
European Alliance for Medical and Biological Engineering & Science
International Union for Physical and Engineering Sciences in Medicine
Intensive Care Unit
Società Italiana di Anestesia Analgesia Rianimazione e Terapia Intensiva
National Health Service
Personal Protective Equipment
French S. Between factualism and substantialism: Structuralism as a third way. Int J Philos Stud. 2018;26(5):701–21.
Article Google Scholar
Morin E. From the concept of system to the paradigm of complexity. J Soc Evolut Syst. 1992;15(4):371–85.
Department for Health of Ireland. Ethical framework for decision-making in a pandemic. https://assets.gov.ie/72072/989943ddd0774e7aa1c01cc9d428b159.pdf (2020). Accessed 19 June 2020.
The Nuffield Council of Bioethics. Responding to the COVID-19 Pandemic: Ethical Considerations. https://www.nuffieldbioethics.org/news/responding-to-the-covid-19-pandemic-ethical-considerations (2020). Accessed 19 June 2020.
Comité De Bioética De España. Informe Del Comité De Bioética De España Sobre Los Aspectos Bioéticos De La Priorización De Recursos Sanitarios En El Contexto De La Crisis Del Coronavirus. http://assets.comitedebioetica.es/files/documentacion/Informe CBE-Priorizacion de recursos sanitarios-coronavirus CBE.pdf (2020). Accessed 19 June 2020.
Deutscher Ethikrat. Solidarity and responsibility during the coronavirus crisis. https://www.ethikrat.org/en/press-releases/2020/solidarity-and-responsibility-during-the-coronavirus-crisis/ (2020). Accessed 19 June 2020.
UNESCO. Ethics in Research in Times of Pandemic COVID-19. https://en.unesco.org/news/ethics-research-times-pandemic-covid-19 (2020). Accessed 19 June 2020.
UNESCO. Statement on COVID-19: ethical considerations from a global perspective. https://unesdoc.unesco.org/ark:/48223/pf0000373115 (2020). Accessed 19 June 2020.
WHO. Ethical standards for research during public health emergencies: distilling existing guidance to support COVID-19 R&D. https://www.who.int/blueprint/priority-diseases/key-action/liverecovery-save-of-ethical-standards-for-research-during-public-health-emergencies.pdf?ua=1 (2020). Accessed 19 June 2020.
Berlinger N, Wynia M, Powell T, Hester DM, Milliken A, Fabi R, et al. Ethical framework for health care institutions responding to novel Coronavirus SARS-CoV-2 (COVID-19) guidelines for institutional ethics services responding to COVID-19. Safeguarding communities, guiding practice, 2; 2020.
Meyfroidt G, Vlieghe E, Biston P, De Decker K, Wittebole X, Collin V, et al. Ethical principles concerning proportionality of critical care during the COVID-19 pandemic: advice by the Belgian Society of IC medicine. Retrieved 2 April 2020. https://www.hartcentrumhasselt.be/professioneel (2020).
Emanuel EJ, Persad G, Upshur R, Thome B, Parker M, Glickman A, et al. Fair allocation of scarce medical resources in the time of Covid-19. Waltham: Mass Medical Soc.; 2020.
Book Google Scholar
Smith MJ, Ahmad A, Arawi T, Dawson A, Emanuel EJ, Garani-Papadatos T, et al. Top five ethical lessons of COVID-19 that the world must learn. Wellcome Open Res. 2021;6:17.
Smith MJ, Upshur RE. Learning lessons from COVID-19 requires recognizing moral failures. J Bioeth Inq. 2020;17(4):563–6.
Smith MJ, Upshur RE, Emanuel EJ. Publication ethics during public health emergencies such as the COVID-19 pandemic. Am J Public Health. 2020;110(7):947–8.
WHO. Resources on ethics and COVID-19. https://www.who.int/ethics/topics/outbreaks-emergencies/covid-19/en/ (2020). Accessed 19 June 2020.
WHO. Addressing human rights as key to the COVID-19 response. https://www.who.int/publications-detail/addressing-human-rights-as-key-to-the-covid-19-response (2020). Accessed 19 June 2020.
Comitato nazionale per la bioetica. Covid 19: clinical decision-making in conditions of resource shortage and the "pandemic emergency triage criterion". http://bioetica.governo.it/media/3987/p136_2020_covid-19-la-decisione-clinica-in-condizioni-di-carenza-di-risorse-e-il-criterio-del-triage-in-emergenza-pandemica.pdf (2020). Accessed 19 June 2020.
WHO Preparedness. https://www.who.int/environmental_health_emergencies/preparedness/en/ (2020). Accessed 19 June 2020.
The only cure is solidarity from Jürgen Habermas. https://www.classlifestyle.com/news/42575/kura-e-vetme-eshte-solidariteti-nga-jrgen-habermas/eng (2020). Accessed 19 June 2020.
Vergano M, Bertolini G, Giannini A, Gristina GR, Livigni S, Mistraletti G, et al. Clinical ethics recommendations for the allocation of intensive care treatments in exceptional, resource-limited circumstances: the Italian perspective during the COVID-19 epidemic. Berlin: Springer; 2020.
Google Scholar
Rosenbaum L. Facing COVID-19 in Italy—ethics, logistics, and therapeutics on the epidemic’s front line. N Engl J Med. 2020;382(20):1873–5.
Nicoli F, Gasparetto A. Italy in a time of emergency and scarce resources: the need for embedding ethical reflection in social and clinical settings. J Clin Ethics. 2020;31(1):92–4.
Ranney ML, Griffeth V, Jha AK. Critical supply shortages—the need for ventilators and personal protective equipment during the COVID-19 pandemic. N Engl J Med. 2020;382(18):e41.
Sokolov P, Ivanova J. 17th century political cartesianism and its opponents, or imaging the state from point fixe. Higher School of Economics Research Paper No. BRP, 8. 2012.
Jones D. Censorship: a world encyclopedia. London: Routledge; 2001.
Maasen S, Weingart P. Democratization of expertise? Exploring novel forms of scientific advice in political decision-making, vol. 24. Berlin: Springer; 2006.
Bennett B. Logically fallacious: the ultimate collection of over 300 logical fallacies (Academic Edition). EBookIt.com. 2017.
Regulation EU 2017/745 of the European Parliament and of The Council of 5 April 2017 on medical devices, amending Directive 2001/83/EC, Regulation (EC) No 178/2002 and Regulation (EC) No 1223/2009 and repealing Council Directives 90/385/EEC and 93/42/EEC (2017). In: The European parliament and the council of the European Union (Ed.).
Jonas H. The imperative of responsibility: In search of an ethics for the technological age. 1984.
Bentham J, Mill JS. Utilitarianism and other essays. London: Penguin; 2004.
Dewey J. The essential Dewey: pragmatism, education, democracy, vol. 1. Bloomington: Indiana University Press; 1998.
WHO. Novel coronavirus (2019-nCoV) Situation Report-13; 2020.
Pecchia L, Piaggio D, Maccaro A, Formisano C, Iadanza E. The inadequacy of regulatory frameworks in time of crisis and in low-resource settings: personal protective equipment and COVID-19. Health Technol. 2020;10:1375–83.
WHO. Rational use of personal protective equipment for COVID-19 and considerations during severe shortages: interim guidance, 23 December 2020. World Health Organization; 2020.
WHO. Technical specifications of personal protective equipment for COVID-19.
Fletcher JF. Situation ethics: the new morality. Louisville: Westminster John Knox Press; 1997.
Maccaro A, Piaggio D, Pagliara S, Pecchia L. The role of ethics in science: a systematic literature review from the first wave of COVID-19. Health Technol. 2021;3:1–9.
Download references
Acknowledgements
The authors would like to acknowledge Hardip Boparai (PhD student at the University of Warwick, UK) and Katy Stokes (PhD student at the University of Warwick, UK) for proofreading this manuscript and for their valuable suggestions.
DP and LP received support from the University of Warwick with two Warwick Impact Found Grants supported by the EPSRC Impact Accelerator Award (EP/K503848/1 and EP/R511808/1). AM’s Fellowship is supported by the WIRL COFUND – Marie Sklodowska Curie Actions, Institute of Advanced Study, University of Warwick (UK). DP, LP, and AM also received support from Health Global Research Priorities of the University of Warwick. By supporting the researchers, the funders indirectly supported all the parts of this study.
Author information
Alessia Maccaro and Davide Piaggio contributed equally to this work.
Authors and Affiliations
School of Engineering, University of Warwick, Coventry, CV47AL, UK
Alessia Maccaro, Davide Piaggio & Leandro Pecchia
Institute of Advanced Study, University of Warwick, Coventry, CV47AL, UK
Alessia Maccaro
Department of Advanced Biomedical Sciences, University of Naples Federico II, Naples, Italy
Concetta Anna Dodaro
European Alliance of Medical and Biological Engineering and Science (EAMBES), Leuven, Belgium
Leandro Pecchia
IUPESM, York, UK
You can also search for this author in PubMed Google Scholar
Contributions
Conceptualization (AM and LP), data curation (AM, DP), Funding acquisition (LP), investigation (AM, DP, CAD), Project administration (LP), Supervision (LP, CAD), Writing original draft (AM, DP), Writing review and editing (AM, DP, LP, CAD). All authors reviewed the manuscript and agreed with the final version.
Corresponding author
Correspondence to Davide Piaggio .
Ethics declarations
Ethics approval and consent to participate, consent for publication, competing interests.
The authors declare that they have no conflict of interest.
Additional information
Publisher's note.
Springer Nature remains neutral with regard to jurisdictional claims in published maps and institutional affiliations.
Rights and permissions
Open Access This article is licensed under a Creative Commons Attribution 4.0 International License, which permits use, sharing, adaptation, distribution and reproduction in any medium or format, as long as you give appropriate credit to the original author(s) and the source, provide a link to the Creative Commons licence, and indicate if changes were made. The images or other third party material in this article are included in the article's Creative Commons licence, unless indicated otherwise in a credit line to the material. If material is not included in the article's Creative Commons licence and your intended use is not permitted by statutory regulation or exceeds the permitted use, you will need to obtain permission directly from the copyright holder. To view a copy of this licence, visit http://creativecommons.org/licenses/by/4.0/ . The Creative Commons Public Domain Dedication waiver ( http://creativecommons.org/publicdomain/zero/1.0/ ) applies to the data made available in this article, unless otherwise stated in a credit line to the data.
Reprints and permissions
About this article
Cite this article.
Maccaro, A., Piaggio, D., Dodaro, C.A. et al. Biomedical engineering and ethics: reflections on medical devices and PPE during the first wave of COVID-19. BMC Med Ethics 22 , 130 (2021). https://doi.org/10.1186/s12910-021-00697-1
Download citation
Received : 17 August 2020
Accepted : 12 July 2021
Published : 25 September 2021
DOI : https://doi.org/10.1186/s12910-021-00697-1
Share this article
Anyone you share the following link with will be able to read this content:
Sorry, a shareable link is not currently available for this article.
Provided by the Springer Nature SharedIt content-sharing initiative
- Biomedical engineering
- Regulations
- Responsibility
BMC Medical Ethics
ISSN: 1472-6939
- General enquiries: [email protected]

Health Care Comes Home: The Human Factors (2011)
Chapter: 7 conclusions and recommendations.
7 Conclusions and Recommendations
Health care is moving into the home increasingly often and involving a mixture of people, a variety of tasks, and a broad diversity of devices and technologies; it is also occurring in a range of residential environments. The factors driving this migration include the rising costs of providing health care; the growing numbers of older adults; the increasing prevalence of chronic disease; improved survival rates of various diseases, injuries, and other conditions (including those of fragile newborns); large numbers of veterans returning from war with serious injuries; and a wide range of technological innovations. The health care that results varies considerably in its safety, effectiveness, and efficiency, as well as its quality and cost.
The committee was charged with examining this major trend in health care delivery and resulting challenges from only one of many perspectives: the study of human factors. From the outset it was clear that the dramatic and evolving change in health care practice and policies presents a broad array of opportunities and problems. Consequently the committee endeavored to maintain focus specifically on how using the human factors approach can provide solutions that support maximizing the safety and quality of health care delivered in the home while empowering both care recipients and caregivers in the effort.
The conclusions and recommendations presented below reflect the most critical steps that the committee thinks should be taken to improve the state of health care in the home, based on the literature reviewed in this report examined through a human factors lens. They are organized into four areas: (1) health care technologies, including medical devices and health information technologies involved in health care in the home; (2)
caregivers and care recipients; (3) residential environments for health care; and (4) knowledge gaps that require additional research and development. Although many issues related to home health care could not be addressed, applications of human factors principles, knowledge, and research methods in these areas could make home health care safer and more effective and also contribute to reducing costs. The committee chose not to prioritize the recommendations, as they focus on various aspects of health care in the home and are of comparable importance to the different constituencies affected.
HEALTH CARE TECHNOLOGIES
Health care technologies include medical devices that are used in the home as well as information technologies related to home-based health care. The four recommendations in this area concern (1) regulating technologies for health care consumers, (2) developing guidance on the structure and usability of health information technologies, (3) developing guidance and standards for medical device labeling, and (4) improving adverse event reporting systems for medical devices. The adoption of these recommendations would improve the usability and effectiveness of technology systems and devices, support users in understanding and learning to use them, and improve feedback to government and industry that could be used to further improve technology for home care.
Ensuring the safety of emerging technologies is a challenge, in part because it is not always clear which federal agency has regulatory authority and what regulations must be met. Currently, the U.S. Food and Drug Administration (FDA) has responsibility for devices, and the Office of the National Coordinator for Health Information Technology (ONC) has similar authority with respect to health information technology. However, the dividing line between medical devices and health information technology is blurring, and many new systems and applications are being developed that are a combination of the two, although regulatory oversight has remained divided. Because regulatory responsibility for them is unclear, these products may fall into the gap.
The committee did not find a preponderance of evidence that knowledge is lacking for the design of safe and effective devices and technologies for use in the home. Rather than discovering an inadequate evidence base, we were troubled by the insufficient attention directed at the development of devices that account, necessarily and properly, for users who are inadequately trained or not trained at all. Yet these new users often must
rely on equipment without ready knowledge about limitations, maintenance requirements, and problems with adaptation to their particular home settings.
The increased prominence of the use of technology in the health care arena poses predictable challenges for many lay users, especially people with low health literacy, cognitive impairment, or limited technology experience. For example, remote health care management may be more effective when it is supported by technology, and various electronic health care (“e-health”) applications have been developed for this purpose. With the spectrum of caregivers ranging from individuals caring for themselves or other family members to highly experienced professional caregivers, computer-based care management systems could offer varying levels of guidance, reminding, and alerting, depending on the sophistication of the operator and the criticality of the message. However, if these technologies or applications are difficult to understand or use, they may be ignored or misused, with potentially deleterious effects on care recipient health and safety. Applying existing accessibility and usability guidelines and employing user-centered design and validation methods in the development of health technology products designed for use in the home would help ensure that they are safe and effective for their targeted user populations. In this effort, it is important to recognize how the line between medical devices and health information technologies has become blurred while regulatory oversight has remained distinct, and it is not always clear into which domain a product falls.
Recommendation 1. The U.S. Food and Drug Administration and the Office of the National Coordinator for Health Information Technology should collaborate to regulate, certify, and monitor health care applications and systems that integrate medical devices and health information technologies. As part of the certification process, the agencies should require evidence that manufacturers have followed existing accessibility and usability guidelines and have applied user-centered design and validation methods during development of the product.
Guidance and Standards
Developers of information technologies related to home-based health care, as yet, have inadequate or incomplete guidance regarding product content, structure, accessibility, and usability to inform innovation or evolution of personal health records or of care recipient access to information in electronic health records.
The ONC, in the initial announcement of its health information technology certification program, stated that requirements would be forthcom-
ing with respect both to personal health records and to care recipient access to information in electronic health records (e.g., patient portals). Despite the importance of these requirements, there is still no guidance on the content of information that should be provided to patients or minimum standards for accessibility, functionality, and usability of that information in electronic or nonelectronic formats.
Consequently, some portals have been constructed based on the continuity of care record. However, recent research has shown that records and portals based on this model are neither understandable nor interpretable by laypersons, even by those with a college education. The lack of guidance in this area makes it difficult for developers of personal health records and patient portals to design systems that fully address the needs of consumers.
Recommendation 2. The Office of the National Coordinator for Health Information Technology, in collaboration with the National Institute of Standards and Technology and the Agency for Healthcare Research and Quality, should establish design guidelines and standards, based on existing accessibility and usability guidelines, for content, accessibility, functionality, and usability of consumer health information technologies related to home-based health care.
The committee found a serious lack of adequate standards and guidance for the labeling of medical devices. Furthermore, we found that the approval processes of the FDA for changing these materials are burdensome and inflexible.
Just as many medical devices currently in use by laypersons in the home were originally designed and approved for use only by professionals in formal health care facilities, the instructions for use and training materials were not designed for lay users, either. The committee recognizes that lack of instructional materials for lay users adds to the level of risk involved when devices are used by populations for whom they were not intended.
Ironically, the FDA’s current premarket review and approval processes inadvertently discourage manufacturers from selectively revising or developing supplemental instructional and training materials, when they become aware that instructional and training materials need to be developed or revised for lay users of devices already approved and marketed. Changing the instructions for use (which were approved with the device) requires manufacturers to submit the device along with revised instructions to the FDA for another 510(k) premarket notification review. Since manufacturers can find these reviews complicated, time-consuming, and expensive, this requirement serves as a disincentive to appropriate revisions of instructional or training materials.
Furthermore, little guidance is currently available on design of user
training methods and materials for medical devices. Even the recently released human factors standard on medical device design (Association for the Advancement of Medical Instrumentation, 2009), while reasonably comprehensive, does not cover the topic of training or training materials. Both FDA guidance and existing standards that do specifically address the design of labeling and ensuing instructions for use fail to account for up-to-date findings from research on instructional systems design. In addition, despite recognition that requirements for user training, training materials, and instructions for use are different for lay and professional users of medical equipment, these differences are not reflected in current standards.
Recommendation 3. The U.S. Food and Drug Administration (FDA) should promote development (by standards development organizations, such as the International Electrotechnical Commission, the International Organization for Standardization, the American National Standards Institute, and the Association for the Advancement of Medical Instrumentation) of new standards based on the most recent human factors research for the labeling of and ensuing instructional materials for medical devices designed for home use by lay users. The FDA should also tailor and streamline its approval processes to facilitate and encourage regular improvements of these materials by manufacturers.
Adverse Event Reporting Systems
The committee notes that the FDA’s adverse event reporting systems, used to report problems with medical devices, are not user-friendly, especially for lay users, who generally are not aware of the systems, unaware that they can use them to report problems, and uneducated about how to do so. In order to promote safe use of medical devices in the home and rectify design problems that put care recipients at risk, it is necessary that the FDA conduct more effective postmarket surveillance of medical devices to complement its premarket approval process. The most important elements of their primarily passive surveillance system are the current adverse event reporting mechanisms, including Maude and MedSun. Entry of incident data by health care providers and consumers is not straightforward, and the system does not elicit data that could be useful to designers as they develop updated versions of products or new ones that are similar to existing devices. The reporting systems and their importance need to be widely promoted to a broad range of users, especially lay users.
Recommendation 4. The U.S. Food and Drug Administration should improve its adverse event reporting systems to be easier to use, to collect data that are more useful for identifying the root causes of events
related to interactions with the device operator, and to develop and promote a more convenient way for lay users as well as professionals to report problems with medical devices.
CAREGIVERS IN THE HOME
Health care is provided in the home by formal caregivers (health care professionals), informal caregivers (family and friends), and individuals who self-administer care; each type of caregiver faces unique issues. Properly preparing individuals to provide care at home depends on targeting efforts appropriately to the background, experience, and knowledge of the caregivers. To date, however, home health care services suffer from being organized primarily around regulations and payments designed for inpatient or outpatient acute care settings. Little attention has been given to how different the roles are for formal caregivers when delivering services in the home or to the specific types of training necessary for appropriate, high-quality practice in this environment.
Health care administration in the home commonly involves interaction among formal caregivers and informal caregivers who share daily responsibility for a person receiving care. But few formal caregivers are given adequate training on how to work with informal caregivers and involve them effectively in health decision making, use of medical or adaptive technologies, or best practices to be used for evaluating and supporting the needs of caregivers.
It is also important to recognize that the majority of long-term care provided to older adults and individuals with disabilities relies on family members, friends, or the individual alone. Many informal caregivers take on these responsibilities without necessary education or support. These individuals may be poorly prepared and emotionally overwhelmed and, as a result, experience stress and burden that can lead to their own morbidity. The committee is aware that informational and training materials and tested programs already exist to assist informal caregivers in understanding the many details of providing health care in the home and to ease their burden and enhance the quality of life of both caregiver and care recipient. However, tested materials and education, support, and skill enhancement programs have not been adequately disseminated or integrated into standard care practices.
Recommendation 5. Relevant professional practice and advocacy groups should develop appropriate certification, credentialing, and/or training standards that will prepare formal caregivers to provide care in the home, develop appropriate informational and training materials
for informal caregivers, and provide guidance for all caregivers to work effectively with other people involved.
RESIDENTIAL ENVIRONMENTS FOR HEALTH CARE
Health care is administered in a variety of nonclinical environments, but the most common one, particularly for individuals who need the greatest level and intensity of health care services, is the home. The two recommendations in this area encourage (1) modifications to existing housing and (2) accessible and universal design of new housing. The implementation of these recommendations would be a good start on an effort to improve the safety and ease of practicing health care in the home. It could improve the health and safety of many care recipients and their caregivers and could facilitate adherence to good health maintenance and treatment practices. Ideally, improvements to housing design would take place in the context of communities that provide transportation, social networking and exercise opportunities, and access to health care and other services.
Safety and Modification of Existing Housing
The committee found poor appreciation of the importance of modifying homes to remove health hazards and barriers to self-management and health care practice and, furthermore, that financial support from federal assistance agencies for home modifications is very limited. The general connection between housing characteristics and health is well established. For example, improving housing conditions to enhance basic sanitation has long been part of a public health response to acute illness. But the characteristics of the home can present significant barriers to autonomy or self-care management and present risk factors for poor health, injury, compromised well-being, and greater dependence on others. Conversely, physical characteristics of homes can enhance resident safety and ability to participate in daily self-care and to utilize effectively health care technologies that are designed to enhance health and well-being.
Home modifications based on professional home assessments can increase functioning, contribute to reducing accidents such as falls, assist caregivers, and enable chronically ill persons and people with disabilities to stay in the community. Such changes are also associated with facilitating hospital discharges, decreasing readmissions, reducing hazards in the home, and improving care coordination. Familiar modifications include installation of such items as grab bars, handrails, stair lifts, increased lighting, and health monitoring equipment as well as reduction of such hazards as broken fixtures and others caused by insufficient home maintenance.
Deciding on which home modifications have highest priority in a given
setting depends on an appropriate assessment of circumstances and the environment. A number of home assessment instruments and programs have been validated and proven to be effective to meet this need. But even if needed modifications are properly identified and prioritized, inadequate funding, gaps in services, and lack of coordination between the health and housing service sectors have resulted in a poorly integrated system that is difficult to access. Even when accessed, progress in making home modifications available has been hampered by this lack of coordination and inadequate reimbursement or financial mechanisms, especially for those who cannot afford them.
Recommendation 6. Federal agencies, including the U.S. Department of Health and Human Services and the Centers for Medicare & Medicaid Services, along with the U.S. Department of Housing and Urban Development and the U.S. Department of Energy, should collaborate to facilitate adequate and appropriate access to health- and safety-related home modifications, especially for those who cannot afford them. The goal should be to enable persons whose homes contain obstacles, hazards, or features that pose a home safety concern, limit self-care management, or hinder the delivery of needed services to obtain home assessments, home modifications, and training in their use.
Accessibility and Universal Design of New Housing
Almost all existing housing in the United States presents problems for conducting health-related activities because physical features limit independent functioning, impede caregiving, and contribute to such accidents as falls. In spite of the fact that a large and growing number of persons, including children, adults, veterans, and older adults, have disabilities and chronic conditions, new housing continues to be built that does not account for their needs (current or future). Although existing homes can be modified to some extent to address some of the limitations, a proactive, preventive, and effective approach would be to plan to address potential problems in the design phase of new and renovated housing, before construction.
Some housing is already required to be built with basic accessibility features that facilitate practice of health care in the home as a result of the Fair Housing Act Amendments of 1998. And 17 states and 30 cities have passed what are called “visitability” codes, which currently apply to 30,000 homes. Some localities offer tax credits, such as Pittsburgh through an ordinance, to encourage installing visitability features in new and renovated housing. The policy in Pittsburgh was impetus for the Pennsylvania Residential VisitAbility Design Tax Credit Act signed into law on October 28, 2006, which offers property owners a tax credit for new construction
and rehabilitation. The Act paves the way for municipalities to provide tax credits to citizens by requiring that such governing bodies administer the tax credit (Self-Determination Housing Project of Pennsylvania, Inc., n.d.).
Visitability, rather than full accessibility, is characterized by such limited features as an accessible entry into the home, appropriately wide doorways and one accessible bathroom. Both the International Code Council, which focuses on building codes, and the American National Standards Institute, which establishes technical standards, including ones associated with accessibility, have endorsed voluntary accessibility standards. These standards facilitate more jurisdictions to pass such visitability codes and encourage legislative consistency throughout the country. To date, however, the federal government has not taken leadership to promote compliance with such standards in housing construction, even for housing for which it provides financial support.
Universal design, a broader and more comprehensive approach than visitability, is intended to suit the needs of persons of all ages, sizes, and abilities, including individuals with a wide range of health conditions and activity limitations. Steps toward universal design in renovation could include such features as anti-scald faucet valve devices, nonslip flooring, lever handles on doors, and a bedroom on the main floor. Such features can help persons and their caregivers carry out everyday tasks and reduce the incidence of serious and costly accidents (e.g., falls, burns). In the long run, implementing universal design in more homes will result in housing that suits the long-term needs of more residents, provides more housing choices for persons with chronic conditions and disabilities, and causes less forced relocation of residents to more costly settings, such as nursing homes.
Issues related to housing accessibility have been acknowledged at the federal level. For example, visitability and universal design are in accord with the objectives of the Safety of Seniors Act (Public Law No. 110-202, passed in 2008). In addition, implementation of the Olmstead decision (in which the U.S. Supreme Court ruled that the Americans with Disabilities Act may require states to provide community-based services rather than institutional placements for individuals with disabilities) requires affordable and accessible housing in the community.
Visitability, accessibility, and universal design of housing all are important to support the practice of health care in the home, but they are not broadly implemented and incentives for doing so are few.
Recommendation 7. Federal agencies, such as the U.S. Department of Housing and Urban Development, the U.S. Department of Veterans Affairs, and the Federal Housing Administration, should take a lead role, along with states and local municipalities, to develop strategies that promote and facilitate increased housing visitability, accessibil-
ity, and universal design in all segments of the market. This might include tax and other financial incentives, local zoning ordinances, model building codes, new products and designs, and related policies that are developed as appropriate with standards-setting organizations (e.g., the International Code Council, the International Electrotechnical Commission, the International Organization for Standardization, and the American National Standards Institute).
RESEARCH AND DEVELOPMENT
In our review of the research literature, the committee learned that there is ample foundational knowledge to apply a human factors lens to home health care, particularly as improvements are considered to make health care safe and effective in the home. However, much of what is known is not being translated effectively into practice, neither in design of equipment and information technology or in the effective targeting and provision of services to all those in need. Consequently, the four recommendations that follow support research and development to address knowledge and communication gaps and facilitate provision of high-quality health care in the home. Specifically, the committee recommends (1) research to enhance coordination among all the people who play a role in health care practice in the home, (2) development of a database of medical devices in order to facilitate device prescription, (3) improved surveys of the people involved in health care in the home and their residential environments, and (4) development of tools for assessing the tasks associated with home-based health care.
Health Care Teamwork and Coordination
Frail elders, adults with disabilities, disabled veterans, and children with special health care needs all require coordination of the care services that they receive in the home. Home-based health care often involves a large number of elements, including multiple care providers, support services, agencies, and complex and dynamic benefit regulations, which are rarely coordinated. However, coordinating those elements has a positive effect on care recipient outcomes and costs of care. When successful, care coordination connects caregivers, improves communication among caregivers and care recipients and ensures that receivers of care obtain appropriate services and resources.
To ensure safe, effective, and efficient care, everyone involved must collaborate as a team with shared objectives. Well-trained primary health care teams that execute customized plans of care are a key element of coordinated care; teamwork and communication among all actors are also
essential to successful care coordination and the delivery of high-quality care. Key factors that influence the smooth functioning of a team include a shared understanding of goals, common information (such as a shared medication list), knowledge of available resources, and allocation and coordination of tasks conducted by each team member.
Barriers to coordination include insufficient resources available to (a) help people who need health care at home to identify and establish connections to appropriate sources of care, (b) facilitate communication and coordination among caregivers involved in home-based health care, and (c) facilitate communication among the people receiving and the people providing health care in the home.
The application of systems analysis techniques, such as task analysis, can help identify problems in care coordination systems and identify potential intervention strategies. Human factors research in the areas of communication, cognitive aiding and decision support, high-fidelity simulation training techniques, and the integration of telehealth technologies could also inform improvements in care coordination.
Recommendation 8 . The Agency for Healthcare Research and Quality should support human factors–based research on the identified barriers to coordination of health care services delivered in the home and support user-centered development and evaluation of programs that may overcome these barriers.
Medical Device Database
It is the responsibility of physicians to prescribe medical devices, but in many cases little information is readily available to guide them in determining the best match between the devices available and a particular care recipient. No resource exists for medical devices, in contrast to the analogous situation in the area of assistive and rehabilitation technologies, for which annotated databases (such as AbleData) are available to assist the provider in determining the most appropriate one of several candidate devices for a given care recipient. Although specialists are apt to receive information about devices specific to the area of their practice, this is much less likely in the case of family and general practitioners, who often are responsible for selecting, recommending, or prescribing the most appropriate device for use at home.
Recommendation 9. The U.S. Food and Drug Administration, in collaboration with device manufacturers, should establish a medical device database for physicians and other providers, including pharmacists, to use when selecting appropriate devices to prescribe or recommend
for people receiving or self-administering health care in the home. Using task analysis and other human factors approaches to populate the medical device database will ensure that it contains information on characteristics of the devices and implications for appropriate care recipient and device operator populations.
Characterizing Caregivers, Care Recipients, and Home Environments
As delivery of health care in the home becomes more common, more coherent strategies and effective policies are needed to support the workforce of individuals who provide this care. Developing these will require a comprehensive understanding of the number and attributes of individuals engaged in health care in the home as well as the context in which care is delivered. Data and data analysis are lacking to accomplish this objective.
National data regarding the numbers of individuals engaged in health care delivery in the home—that is, both formal and informal caregivers—are sparse, and the estimates that do exist vary widely. Although the Bureau of Labor Statistics publishes estimates of the number of workers employed in the home setting for some health care classifications, they do not include all relevant health care workers. For example, data on workers employed directly by care recipients and their families are notably absent. Likewise, national estimates of the number of informal caregivers are obtained from surveys that use different methodological approaches and return significantly different results.
Although numerous national surveys have been designed to answer a broad range of questions regarding health care delivery in the home, with rare exceptions such surveys reflect the relatively limited perspective of the sponsoring agency. For example,
- The Medicare Current Beneficiary Survey (administered by the Centers for Medicare & Medicaid Services) and the Health and Retirement Survey (administered by the National Institute on Aging) are primarily geared toward understanding the health, health services use, and/or economic well-being of older adults and provide no information regarding working-age adults or children or information about home or neighborhood environments.
- The Behavioral Risk Factors Surveillance Survey (administered by the Centers for Disease Control and Prevention, CDC), the National Health Interview Survey (administered by the CDC), and the National Children’s Study (administered by the U.S. Department of Health and Human Services and the U.S. Environmental Protection Agency) all collect information on health characteristics, with limited or no information about the housing context.
- The American Housing Survey (administered by the U.S. Department of Housing and Urban Development) collects detailed information regarding housing, but it does not include questions regarding the health status of residents and does not collect adequate information about home modifications and features on an ongoing basis.
Consequently, although multiple federal agencies collect data on the sociodemographic and health characteristics of populations and on the nation’s housing stock, none of these surveys collects data necessary to link the home, its residents, and the presence of any caregivers, thus limiting understanding of health care delivered in the home. Furthermore, information is altogether lacking about health and functioning of populations linked to the physical, social, and cultural environments in which they live. Finally, in regard to individuals providing care, information is lacking regarding their education, training, competencies, and credentialing, as well as appropriate knowledge about their working conditions in the home.
Better coordination across government agencies that sponsor such surveys and more attention to information about health care that occurs in the home could greatly improve the utility of survey findings for understanding the prevalence and nature of health care delivery in the home.
Recommendation 10. Federal health agencies should coordinate data collection efforts to capture comprehensive information on elements relevant to health care in the home, either in a single survey or through effective use of common elements across surveys. The surveys should collect data on the sociodemographic and health characteristics of individuals receiving care in the home, the sociodemographic attributes of formal and informal caregivers and the nature of the caregiving they provide, and the attributes of the residential settings in which the care recipients live.
Tools for Assessing Home Health Care Tasks and Operators
Persons caring for themselves or others at home as well as formal caregivers vary considerably in their skills, abilities, attitudes, experience, and other characteristics, such as age, culture/ethnicity, and health literacy. In turn, designers of health-related devices and technology systems used in the home are often naïve about the diversity of the user population. They need high-quality information and guidance to better understand user capabilities relative to the task demands of the health-related device or technology that they are developing.
In this environment, valid and reliable tools are needed to match users with tasks and technologies. At this time, health care providers lack the
tools needed to assess whether particular individuals would be able to perform specific health care tasks at home, and medical device and system designers lack information on the demands associated with health-related tasks performed at home and the human capabilities needed to perform them successfully.
Whether used to assess the characteristics of formal or informal caregivers or persons engaged in self-care, task analysis can be used to develop point-of-care tools for use by consumers and caregivers alike in locations where such tasks are encouraged or prescribed. The tools could facilitate identification of potential mismatches between the characteristics, abilities, experiences, and attitudes that an individual brings to a task and the demands associated with the task. Used in ambulatory care settings, at hospital discharge or other transitions of care, and in the home by caregivers or individuals and family members themselves, these tools could enable assessment of prospective task performer’s capabilities in relation to the demands of the task. The tools might range in complexity from brief screening checklists for clinicians to comprehensive assessment batteries that permit nuanced study and tracking of home-based health care tasks by administrators and researchers. The results are likely to help identify types of needed interventions and support aids that would enhance the abilities of individuals to perform health care tasks in home settings safely, effectively, and efficiently.
Recommendation 11. The Agency for Healthcare Research and Quality should collaborate, as necessary, with the National Institute for Disability and Rehabilitation Research, the National Institutes of Health, the U.S. Department of Veterans Affairs, the National Science Foundation, the U.S. Department of Defense, and the Centers for Medicare & Medicaid Services to support development of assessment tools customized for home-based health care, designed to analyze the demands of tasks associated with home-based health care, the operator capabilities required to carry them out, and the relevant capabilities of specific individuals.
Association for the Advancement of Medical Instrumentation. (2009). ANSI/AAMI HE75:2009: Human factors engineering: Design of medical devices. Available: http://www.aami.org/publications/standards/HE75_Ch16_Access_Board.pdf [April 2011].
Self-Determination Housing Project of Pennsylvania, Inc. (n.d.) Promoting visitability in Pennsylvania. Available: http://www.sdhp.org/promoting_visitability_in_pennsy.htm [March 30, 2011].
In the United States, health care devices, technologies, and practices are rapidly moving into the home. The factors driving this migration include the costs of health care, the growing numbers of older adults, the increasing prevalence of chronic conditions and diseases and improved survival rates for people with those conditions and diseases, and a wide range of technological innovations. The health care that results varies considerably in its safety, effectiveness, and efficiency, as well as in its quality and cost.
Health Care Comes Home reviews the state of current knowledge and practice about many aspects of health care in residential settings and explores the short- and long-term effects of emerging trends and technologies. By evaluating existing systems, the book identifies design problems and imbalances between technological system demands and the capabilities of users. Health Care Comes Home recommends critical steps to improve health care in the home. The book's recommendations cover the regulation of health care technologies, proper training and preparation for people who provide in-home care, and how existing housing can be modified and new accessible housing can be better designed for residential health care. The book also identifies knowledge gaps in the field and how these can be addressed through research and development initiatives.
Health Care Comes Home lays the foundation for the integration of human health factors with the design and implementation of home health care devices, technologies, and practices. The book describes ways in which the Agency for Healthcare Research and Quality (AHRQ), the U.S. Food and Drug Administration (FDA), and federal housing agencies can collaborate to improve the quality of health care at home. It is also a valuable resource for residential health care providers and caregivers.
READ FREE ONLINE
Welcome to OpenBook!
You're looking at OpenBook, NAP.edu's online reading room since 1999. Based on feedback from you, our users, we've made some improvements that make it easier than ever to read thousands of publications on our website.
Do you want to take a quick tour of the OpenBook's features?
Show this book's table of contents , where you can jump to any chapter by name.
...or use these buttons to go back to the previous chapter or skip to the next one.
Jump up to the previous page or down to the next one. Also, you can type in a page number and press Enter to go directly to that page in the book.
Switch between the Original Pages , where you can read the report as it appeared in print, and Text Pages for the web version, where you can highlight and search the text.
To search the entire text of this book, type in your search term here and press Enter .
Share a link to this book page on your preferred social network or via email.
View our suggested citation for this chapter.
Ready to take your reading offline? Click here to buy this book in print or download it as a free PDF, if available.
Get Email Updates
Do you enjoy reading reports from the Academies online for free ? Sign up for email notifications and we'll let you know about new publications in your areas of interest when they're released.
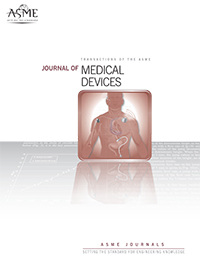
- About the Journal
The Journal of Medical Devices is a unique international forum for rapid publication of significant research on the development and characterization of devices including instruments aimed for biomedical applications. Read more...
Xiaoming He and Carl Nelson , University of Maryland and University of Nebraska-Lincoln View Full Editorial Board

The Spinebot—A Robotic Device to Intraoperatively Quantify Spinal Stiffness
Philippe Büchler, Jonas Räber, Benjamin Voumard, Steve Berger, Brett Bell, Nino Sutter, Stefan Funariu, Carol Hasler, Daniel Studer
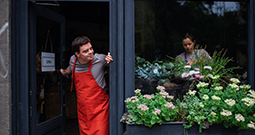
Open Access
The ASME Open Journal of Engineering (AOJE) is a rapid turnaround, multidisciplinary, open access, and rigorously peer-reviewed publication that expands the ASME Journal Program to offer original research across the broad spectrum of all ASME technical communities.
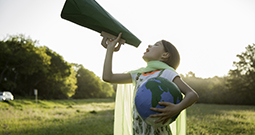
Announcements and Call For Papers
ASME continuously publishes Special Issues in emerging areas. Stay up to date on the latest call for papers if you’d like to submit.
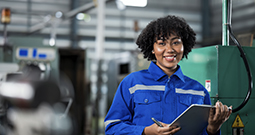
Why Publish in ASME Journals?
Here are 10 important reasons to choose ASME. Become part of the ASME author community and its more than 140-year tradition of promoting the art, science, and practice of multidisciplinary engineering and the related sciences around the globe.
Twitter: @ASMEjournals
Affiliations.
- Accepted Manuscripts
- Editorial Board
- Information for Authors
- Call for Papers
- Rights and Permission
- Online ISSN 1932-619X
- Print ISSN 1932-6181
ASME Journals
- About ASME Journals
- Submit a Paper
- Title History
ASME Conference Proceedings
- About ASME Conference Publications and Proceedings
- Conference Proceedings Author Guidelines
ASME eBooks
- About ASME eBooks
- ASME Press Advisory & Oversight Committee
- Book Proposal Guidelines
- Frequently Asked Questions
- Publication Permissions & Reprints
- ASME Membership
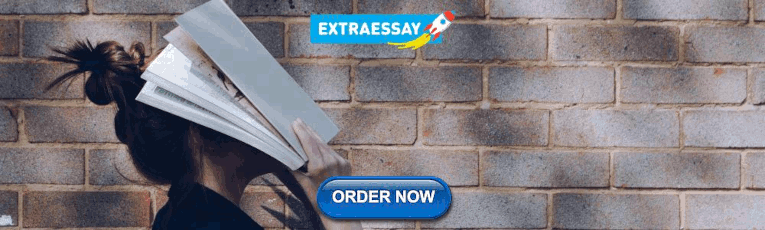
Opportunities
- Faculty Positions
- ASME Community
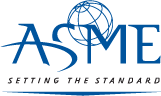
- Accessibility
- Privacy Statement
- Terms of Use
- Get Adobe Acrobat Reader
This Feature Is Available To Subscribers Only
Sign In or Create an Account
Medical Devices Essays
Innovations in audiology: oticon and cochlear americas, a critical analysis of ethical issues in medical devices and instruments, popular essay topics.
- American Dream
- Artificial Intelligence
- Black Lives Matter
- Bullying Essay
- Career Goals Essay
- Causes of the Civil War
- Child Abusing
- Civil Rights Movement
- Community Service
- Cultural Identity
- Cyber Bullying
- Death Penalty
- Depression Essay
- Domestic Violence
- Freedom of Speech
- Global Warming
- Gun Control
- Human Trafficking
- I Believe Essay
- Immigration
- Importance of Education
- Israel and Palestine Conflict
- Leadership Essay
- Legalizing Marijuanas
- Mental Health
- National Honor Society
- Police Brutality
- Pollution Essay
- Racism Essay
- Romeo and Juliet
- Same Sex Marriages
- Social Media
- The Great Gatsby
- The Yellow Wallpaper
- Time Management
- To Kill a Mockingbird
- Violent Video Games
- What Makes You Unique
- Why I Want to Be a Nurse
- Send us an e-mail
Medical Device Development and Design: A Definitive Guide
Table of Contents
Shrinidh Joshi , freelance medical device consultant on Kolabtree, provides a definitive guide to medical device development and design, stressing on important things to keep in mind to make the process smoother and easier.
Before we embark on the journey of medical device development as you might know that developing a medical device is a long and complex process. During recent times, tightening regulatory requirements globally from agencies such as the FDA and EU mean this already expensive process is becoming even more costly. Badly run development projects can overrun on cost and time and may ultimately have to be aborted with huge financial and opportunity costs. For small businesses and start-ups, this can threaten the future of the whole company. Agile development methodology which is more popular with tech startup doesn’t necessarily work in the medical device area where regulation requires all the details and documents in place before a product can be released on the market or tested with users.
Human factors and usability are very important and bring value to medical device development. During the COVID-19 pandemic, many medical device startups are trying to rush to the market by asking a simple question to the regulatory agencies, “We’ve got a working product, can we just get the expedited regulatory approval?”. FDA and other regulatory agencies throughout the world have responded to this unprecedented need by launching programs such as Emergency Use Authorization (EUA ). However, in many cases, the review of the regulatory requirements quickly identifies that a fundamental redesign of a medical device is required, and medical device startups realize that much of the development must be redone.
While Medical Device Regulations aren’t perfect in any jurisdiction, most regulatory authorities intentionally develop regulations that are flexible, performance-based, and open to interpretation. This way they continue to adapt over time, applying to a broad range of technologies and uses. Medical device startups and small businesses have an opportunity to use this to their advantage once they are adequately oriented to this perspective. So, before embarking on the development of a medical device, it is important to understand the process and the potential pitfalls. I think anyone who wants to succeed in innovative medical device development must learn to enjoy solving complex problems (including regulatory problems), or else they will drown in skepticism and frustration. I hope that sharing my perspective might help. With such a complex process, it is impossible for a single article to cover every aspect of medical device development and since I want to provide you with digestible reads, I will be break this up into a series of 8 articles that will cover the areas where medical device companies frequently have problems. I hope you find them useful, so let’s begin with the introductory article which covers the following topics:
- What does usability mean for medical devices?
- Why are human factors important?
- Common errors while designing a medical device
- What is the biggest mistake you can make in medical device development?
- How to focus on delivery
1. What does usability mean for medical devices?
According to a recent study by Johns Hopkins, more than 250,000 people in the United States die every year because of medical mistakes, making it the third leading cause of death after heart disease and cancer [1] , To err is human: Building a safer health system [2] . According to the WHO (World Health Organization)’s website, similar trends exist for European healthcare systems, with estimates that 8 to 12 percent of hospitalizations involve adverse events and that as many as 18 percent of patients report having experienced a medical error-induced problem [3] . Costs in the UK alone for hospital infection intervention is estimated at £2 million per year. Adverse events over the past two decades have shown disturbing trends in post-market events that are attributable to design issues regarding the user interface (UI) of medical devices. Infusion pumps, automatic electronic defibrillators, ventilators, and combination products such as drug auto-injectors, inhalers, and spacer devices have a history of use-related design problems resulting in overdoses, improper therapy delivery, incorrect diagnoses, ER visits, and dangerous delays in therapy. As part of the systematic process to reduce errors by regulatory bodies, medical device companies in the US and EU have been introduced to the disciplines of Human Factors and Usability Engineering. The usability experience has been applied in the automotive, aerospace, and telecommunications industries for more than 60 years, but has only recently been applied in the medical industry. Human factor and usability focus on the synergy of human operators, or users with systems, and their UIs by applying knowledge of human capabilities and limitations and performing tests and evaluations of user/system performance. Human factors also apply known principles and best practices in the design of displays, controls, and other UI aspects to optimize the use and eliminate or limit use-related risks [4] .
Usability should align with the medical device Product Requirement Specification (PRS). PRS defines everything about your product. It is probably the most important document for your whole development as is involved from the beginning to the end of the development. It’s very important from a regulatory point of view but it is also vital from a commercial and practical point of view. When the product specification is not defined keeping the usability in mind it can become the top cause of project delays (jointly with problems with the team), so getting this right, getting it agreed across the business, and sticking to it, are all critical to enabling the rapid development of a successful new medical device. Usability Standards, IEC 62366 , define it as characteristic of the UI that establishes effectiveness, efficiency, and user satisfaction. Therefore, to minimize user errors and use associated risks, reasonable Usability must be achieved by using the usability engineering (UE) process. (IEC 62366:2007 and A1:2014 ).
According to the FDA, the specific beneficial outcomes of applying human factors/usability engineering to medical devices include:
- Easier-to-use devices,
- Safer connections between device components and accessories (e.g., power cords, leads, tubing, cartridges),
- Easier-to-read controls and displays,
- Better user understanding of the device’s status and operation,
- Better user understanding of a patient’s current medical condition,
- More effective alarm signals,
- Easier device maintenance and repair,
- Reduced user reliance on user manuals,
- Reduced need for user training and retraining,
- Reduced risk of use error,
- Reduced risk of adverse events, and
- Reduced risk of product recalls.
It should be noted that the term Usability engineering and Human factors engineering are treated synonymously by Usability standards, EN 62366:2008 and NF EN 62366-1:2015 [5] . Also as per USA FDA “Introduction to Human Factors in Medical Devices”, the terms “human factors engineering,” “usability engineering and ergonomics” are often used interchangeably for the process utilized to achieve highly usable equipment.
Human Factors, however, is defined as “discipline that seeks to improve human performance in the use of equipment by means of hardware and software design that is compatible with the abilities of the user population” (US FDA, An Introduction to Human Factors in Medical Devices, Dec. 1996) [6] .
In nutshell, product development may involve some level of compromise. To make the most successful product possible, it is essential to define the Usability or ‘Ideal User Experience’. However, owing to commercial or technical limitations, the ideal user experience may not be achievable but by defining the ideal, medical device startup can understand the elements which negatively impact the user experience and ensure that they can get the best possible user experience within the constraints of manufacturing processes, technology, cost, and regulations. This applies throughout the development as decisions about necessary changes are always done keeping in mind what is best for the user. Taking this approach has long term benefits too. Technologies develop and become cheaper over time. Even hugely successful devices get replaced by newer, better versions. Keeping a picture of what the best possible product might look like allows you to identify the opportunities created by new technologies, materials, or processes to rapidly create the next innovation in the market.
2. Why are human factors important in medical device design?
Human factors principles have long been applied in high-hazard industries such as aerospace, nuclear, petrochemical, energy, and transport to minimize potential risks. Increasingly, human factors in the life science industry have become recognized as an important topic. While human factors engineering was previously only accepted as necessary for electro-medical devices with complex user interfaces, it has evolved to become a mandatory design input required for the development of the majority of medical products throughout the globe.
A large number of medical devices are used for critical patient monitoring and errors in use, leading to patient harm, have progressively become the main cause for concern for manufacturers and patients alike. The cause of such errors can often be due to poorly designed device user interfaces, particularly where a complex user system is involved.
Infusion pumps, ventilators, automatic electronic defibrillators, and drug-device combination products (e.g. auto-injectors) are recognized as potentially having user interface-related issues that can result in severe hazards such as overdoses and dangerous delays or difficulties in delivering medication. Medical devices have become more and more diverse in their capabilities and are used with increased frequency in busy environments with new distractions and requirements for specialized training. As patient care evolves and is transferred to private homes or public environments, less skilled or even unskilled users including patients and caretakers must be enabled to safely use these complex devices.
Because of the rising instances of UI-induced adverse events, the FDA has begun to include human factor/ user experience (HF/UE) reviews as a routine part of their pre-market approval process at the Center for Devices and Radiological Health’s (CDRH) Office of Device Evaluation [7] .
Likewise, the international regulatory community has incorporated IEC 62366, Medical devices – Application of usability engineering to medical devices , as a part of the approval process outside the US. Both the FDA HF/UE guidance and IEC 62366 outline a process including activities throughout device development culminating in validation testing with the final UI design in simulated use environments. HF/UE activities can be categorized into three major phases:
- Preliminary analyses;
- User interface design/evaluation;
- Simulated use testing (validation).
Readers are directed to additional resources such as AAMI HE75:2009, Human factors engineering – Design of medical devices , and ISO 14971 [8] .
3. Common errors while designing a medical device
A medical device design can fall away from the path and can go astray when the medical device company forgets to take into account the uncertainty factor. Uncertainty equals risk, so to reduce the risk it is important to identify any areas of uncertainty and get answers as early as possible in the project. The best possible way to do this is to ask the following key questions:
- What things we don’t know yet? and
- What are we planning to do differently than what is currently being commonly done?
As an example, recently one of my clients asked to design an inhaler for respiratory diseases. The client was able to quickly achieve their goal by this strategy of asking important questions. The key here is to start by looking at the vision for the project. Putting the answers to these questions in the top-level vision statement. In the case of that client, this simple exercise gave them the following:
“If they can make an inhaler that can universally fit with most of the drugs available in the market and if it can also replace the existing bulky spacer devices with features to be defined, that can be sold profitably at price to be confirmed and can be available in US market within 12 months then they know they can sell? units per year.”
This raised some key questions.
- Are they certain that respiratory patients (target users) want a device to do this job?
- Would they prefer to continue using a spacer device and current inhaler?
- How much are they prepared to pay for such a device (if they want such a device at all)?
- How easy is it to combine an inhaler and a spacer device?
- Is the technology capable of making a universal inhaler device?
- What is the market size?
So, rather than start designing, the first phase of work for this client was focused on answering these questions which naturally led to a research program with target users and healthcare professionals for planning new developments and initiation of activities for FDA approval. The result was that the current technologies couldn’t reliably deliver more than 5-10percent of the medications to the target areas and even with a spacer device, the best possible dug delivery is not more than 20 percent. The research identified that combining them in a single device along with improvement in the drug delivery aspect can deliver more than 45 percent of the drug to the target region of the lungs and can drastically improve the performance of the device.
In summary, the biggest challenge that can impede your development process can be anything, such as avoiding competitor IP by proper patent searches and reviews, or the key risk areas can be a combination of user, technical, and cost-based.
4. The biggest mistake you can make in medical device development
A typical medical device development may take anything from 1 to 4 years to get from the kick-off to market approval and launch. Reducing the time to market can create a competitive advantage so there is always pressure to go faster and reduce development timescales. New product development cycles can be compressed but it is important not to squeeze out the time for creativity. The concept generation stage is where the product takes shape and innovations and value-added features are created. It is important to allow time for the designers to uncover the real user needs, define the ideal user experience, and create designs that address these needs in the best possible way. This typically takes place in the first month or two of the project and sets the direction for the whole development. Rushing this and making poor decisions or missing opportunities has implications for the rest of the project and the whole life of the product. “If you think good design is expensive – try bad design” To accelerate the development, some other activities can happen in parallel and once there is an agreed product vision and design concept, then the medical device development can proceed at full speed.
Medical device innovation presents two kinds of financial challenges: a.) funding the innovation’s development and b.) figuring out who will pay how much for the product or service it yields. One problem is the long investment time needed for new medical devices ( de-novo or 510 (k) ) that require FDA approval [9] . While venture capitalists backing an IT start-up may be able to get their money out in two to three years, investors in a medical device firm have to wait for one to four years depending upon the class of medical device, even to find out whether a product will be approved for use. Another problem is that many traditional sources of capital aren’t familiar with the health care industry, so it’s difficult to find investors, let alone investors who can provide helpful guidance to the innovator. This funding challenge could affect innovation so a medical device company should identify the resources to meet the financial challenges which can impede the entire development process.
5. How to focus on delivery
Once the product vision is defined, the risks are understood and mitigated and the design concept is visualized and selected, then all stakeholders should commit to the development plan and delivering the agreed product. At this point, all efforts must be focused on all the activities that need to be completed before the product can be launched. The project plan should be revisited and updated regularly and potential causes of delay identified and mitigations planned. Delays to decisions are a very common problem, particularly in small to midsize businesses and startups (even in corporate environments), where scheduling a meeting which all stakeholders can attend can lead to a delay of several weeks. Pre-scheduling meetings months in advance or assigning deputies can limit such delays.
At this stage, the project must become the sole focus for the key members of the team responsible for driving the project forwards. Staff with other day to day activities will invariably get pulled away from the project causing inevitable delays. It is in these stages that it can sometimes be possible to accelerate the plan by adding additional team members. Ideally, these should also be solely focused on new device development. If problems with the development do occur, a dedicated team focused on delivering the project vision will be more committed and better able to create a rapid action plan to resolve the difficulties. A dedicated and motivated team can achieve more in one year than a disparate and distracted team would achieve in two.
In conclusion,
- Usability and human factors have become a vital part of the product development process ensuring medical device ease of use and safety of use.
- Worldwide, regulatory organizations have begun a systematic oversight and review process regarding manufacturer compliance with the usability engineering standard. The FDA pre-market device review process now routinely includes human factors risk assessment and validation testing of the device UI with intended users.
- Innovation, HF, and UE activities should be conducted throughout all phases of device design and development including preliminary task and risk analysis, UI design and evaluation, and final summative validation testing in simulated use.
- The focus should be on asking key questions which can align a medical device company with their vision statement.
In the next article, I will take you on a journey about medical device overview and regulation which is an important pillar of medical device development.
Need help to develop your medical device? Work with freelance medical device consultants on Kolabtree.
Hire the author
REFERENCES AND RESOURCES
- https://www.cnbc.com/2018/02/22/medical-errors-third-leading-cause-of-death-in-america.html?__source=sharebar|email&par=sharebar )
- Kohn, L.T., Corrigan, J.M. and Donaldson, M., (eds.), To err is human: Building a safer health system, (Washington DC, National Academy Press, 2000).
- https://www.who.int/news-room/fact-sheets/detail/patient-safety
- Draft Guidance for Industry and Food and Drug Administration Staff – Applying human factors and usability engineering to optimize medical device design, FDA Center for Devices and Regulatory Health, June 2011
- https://lne-america.com/library/news/medical-device-usability-highlights-of-european-regulations-and-standards
- Medical device use-safety: Incorporating human factors engineering into risk management , FDA Center for Devices and Regulatory Health, July 2000
- https://www.federalregister.gov/documents/2000/07/18/00-18061/guidance-for-industry-and-fda-reviewers-on-medical-device-use-safety-incorporating-human-factors
- https://www.greenlight.guru/glossary/iso-14971
- https://www.yakar-eng.co/2018/04/15/differences-between-510k-and-de-novo-submission/
All articles in this series: Medical Device Development and Design: A Definitive Guide Medical Device Development: 3 Tips for Success Medical Device Design: The Essential, Step-by-Step Guide Medical Device Commercialization: 9 Steps from Sketch to Launch How to Overcome Medical Device Commercialization Challenges Medical Device Launch: Key Steps to Bring Your Product to Market Medical Device Post-Market Surveillance: A Comprehensive Guide
Unlock Corporate Benefits • Secure Payment Assistance • Onboarding Support • Dedicated Account Manager
Sign up with your professional email to avail special advances offered against purchase orders, seamless multi-channel payments, and extended support for agreements.
About Author
Ramya Sriram manages digital content and communications at Kolabtree (kolabtree.com), the world's largest freelancing platform for scientists. She has over a decade of experience in publishing, advertising and digital content creation.
Related Posts
How on-demand fda submission consultants can prove valuable to your business, three ways on-demand medical writers can help your business , fda 510k submissions guide: free kolabtree whitepaper, leave a reply cancel reply.
Save my name, email, and website in this browser for the next time I comment.
Automated page speed optimizations for fast site performance
Medical Implants and Their Effects on Life Quality Essay
Medical conditions are very complex and call for diverse treatment methods and techniques. Advancement in the medical field has introduced the use of medical implants in treatment. Medical implants refer to the tissues or manufactured devices that doctors put on the surface or inside the body of a patient. Normally, doctors use medical implants to replace missing and malfunctioning body parts, deliver medication, monitor body function, and to reinforce body organs and tissues. Individuals use medical implants for health and beauty purposes among others. Since medical implants came into existence, many controversies have emerged. These controversies revolve around the impacts of the medical implants on the health and the quality of life of patients. This paper discusses how medical implants affect the quality of life of patients. It focuses on the use of medical implants as lifesavers. Additionally, it shows how medical implants positively influence the quality of life of patients. Overall, this paper seeks to show that medical implants do more good than harm.
Mainly, medics use medical implants for life-saving purposes. They use medical implants widely in hospitals when treating patients. They use them on individuals with impaired or missing body parts. Recent studies show that a section of the population has medical implants on or in their bodies. For instance, research shows that about 10% of people in the United States have medical implants put inside or on the surface of their bodies. These implants are essential as they help save the lives of the individuals using them. An individual may be missing an essential body part or have one that is malfunctioning. This means that the life of that individual is in danger.
However, doctors can change the situation if they use medical implants on the individual. For example, patients with malfunctioning hearts or those whose heart arteries lack strong enough valves have a short lifespan. However, with the help of biomedical implants such as valves and pacemakers medics can save the lives of the individuals. Also, artificial joints, intraocular lenses, cochlear devices are important medical implants. They are important as they help in providing support to other tissues. Today, domestic, industrial, and road accidents are on the rise. Most of these accidents leave people with missing, weak, or malfunctioning tissues or organs. This puts the lives of these individuals in danger. However, medical implants help to treat these individuals.
Although individuals get medical implants for life-saving purposes, individuals also get implants for entirely different reasons. For instance, some individuals get implants for beauty. Medical implants not only play the role of lifesavers but also the role of improving the quality of life of patients. Medical implants such as breast and cochlear implants improve the quality of life of those individuals who use them. Cochlear implants assist patients with impaired hearing. Once in place, these implants allow individuals to communicate and interact with the environment with more ease. They positively influence the physical functioning of such individuals. As mentioned earlier, there are implants that people use for beauty purposes. Such implants include breast implants among others. These implants are useful as they enhance the beauty of individuals who use them. They add to the looks of the respective individuals. Overall, the importance of medical implants in improving the quality of life of patients physically is evident.
Medical implants also help in improving the psychological state of individuals who use them. Many individuals who have medical conditions tend to have psychological problems. For example, it is common to find that patients suffering from heart conditions will have psychological problems. The patients tend to be anxious, worried, and unsettled. For example, if a patient’s heart completely lacks valves or has weak valves the patient lives in fear of the heart malfunctioning. However, if such a patient gets medical implants she/he will have some hope.
Psychologically, this patient will have a better quality of life as he/she will be less anxious. This case also applies to individuals who use medical implants for beauty. In most instances, people who engage in these activities are either insecure, looking for appreciation, or want to feel better about themselves. Once such individuals get implants, they feel they have achieved something worthwhile. This has a direct impact on their psychological well-being. Medical implants improve the quality of these individuals by integrating their egos. Consequently, their psychological statuses improve a great deal. Therefore, the advantages of using medical implants are obvious.
In general, medical implants play an important role in the medical field. They not only save the lives of patients but also improve the quality of lives of those patients physically and psychologically. Although implants are essential, they have some shortcomings such as surgery failures. Such incidents contribute to the reluctant use of medical implants. However, with several advancements and improvements, the medical implants will be among the best solutions for handling patients who need replacement, addition, or support for their missing, malfunction, or unimpressive body parts.
- Chicago (A-D)
- Chicago (N-B)
IvyPanda. (2020, September 30). Medical Implants and Their Effects on Life Quality. https://ivypanda.com/essays/medical-implants-and-their-effects-on-life-quality/
"Medical Implants and Their Effects on Life Quality." IvyPanda , 30 Sept. 2020, ivypanda.com/essays/medical-implants-and-their-effects-on-life-quality/.
IvyPanda . (2020) 'Medical Implants and Their Effects on Life Quality'. 30 September.
IvyPanda . 2020. "Medical Implants and Their Effects on Life Quality." September 30, 2020. https://ivypanda.com/essays/medical-implants-and-their-effects-on-life-quality/.
1. IvyPanda . "Medical Implants and Their Effects on Life Quality." September 30, 2020. https://ivypanda.com/essays/medical-implants-and-their-effects-on-life-quality/.
Bibliography
IvyPanda . "Medical Implants and Their Effects on Life Quality." September 30, 2020. https://ivypanda.com/essays/medical-implants-and-their-effects-on-life-quality/.
- Cochlear Implants for Children in Saudi Arabia
- Early Cochlear Implantation's Impact on Literacy
- Cochlear Implant Selection Candidacy
- The Effects of Cochlear Implants on Expressive Language
- The Cochlear Implant and the Deaf Communities
- Critical Evaluation of Binaural Hearing Marketing Strategy Cochlear Ltd
- Dental Implants: Early, Immediate and Delayed Loading
- A Research on Cochlear Implant
- What Is Breast Implants and How Are They Used?
- Caries: To Save or to Put Implants?
- Foster-Care Centers and Public Health
- Thalassemia as a Genetic Disorder and Its Management
- The Problems of People with Deafness
- Down Syndrome as the Most Common Genetic Condition in the US
- Interprofessional Teams in National Pain Strategy
We use cookies to enhance our website for you. Proceed if you agree to this policy or learn more about it.
- Essay Database >
- Essays Examples >
- Essay Topics
Essays on Medical Device
5 samples on this topic
Writing piles of Medical Device papers is an immanent part of modern studying, be it in high-school, college, or university. If you can do that unassisted, that's just awesome; yet, other learners might not be that skilled, as Medical Device writing can be quite challenging. The catalog of free sample Medical Device papers offered below was assembled in order to help flunker students rise up to the challenge.
On the one hand, Medical Device essays we present here precisely demonstrate how a really remarkable academic piece of writing should be developed. On the other hand, upon your request and for an affordable price, an expert essay helper with the relevant academic background can put together a high-quality paper example on Medical Device from scratch.
Free Safe Medical Device Act Essay Example
A Summation Of The Safe Medical Devices Act
Good Example Of VA Hospital Article Review
VA Hospital
Free Food And Drug Administration Essay Sample
Course work on health care course work.
Health Care Course Work
275 words = 1 page double-spaced
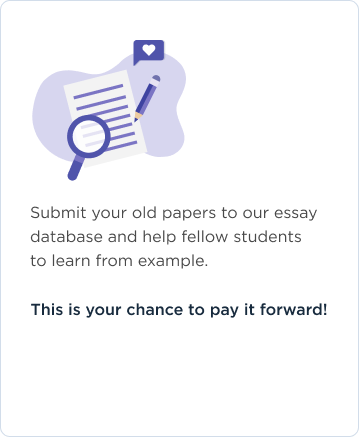
Password recovery email has been sent to [email protected]
Use your new password to log in
You are not register!
By clicking Register, you agree to our Terms of Service and that you have read our Privacy Policy .
Now you can download documents directly to your device!
Check your email! An email with your password has already been sent to you! Now you can download documents directly to your device.
or Use the QR code to Save this Paper to Your Phone
The sample is NOT original!
Short on a deadline?
Don't waste time. Get help with 11% off using code - GETWOWED
No, thanks! I'm fine with missing my deadline

The Role of Regulatory Affairs in the Medical Device Industry
Faculty Insights Industry Advice Regulatory Affairs
Northeastern University lecturer Jeff Shiffman describes the critical role regulatory affairs professionals play in bringing a new medical device to market.
The medical device industry plays a key role in the healthcare ecosystem by providing innovative solutions that improve patient outcomes. Every company seeks to innovate, whether through iterative updates to existing technology or by developing new devices that could offer a more effective treatment to a particular disease or condition.
Regulatory affairs professionals serve a critical function throughout a medical device’s product lifecycle—leading premarket strategy, drafting regulatory submissions , and ensuring postmarket compliance. Here’s a closer look at each of those phases.
Download Our Free Guide to Advancing Your Regulatory Affairs Career
Learn how to navigate the discipline and accelerate your regulatory career.
DOWNLOAD NOW
Regulatory Affairs Responsibilities Throughout the Product Lifecycle
Premarket regulatory strategy.
As product developers work to bring concept to reality, the regulatory affairs professional plays an important role, advising the team on appropriate regulatory strategies to ensure the product can be legally marketed. From the earliest meetings, regulatory affairs must advise the product team on how various decisions may affect the type of regulatory submission required, as well as associated timelines.
For products that will be distributed internationally, regulatory affairs must also coordinate with regional teams to ensure the product has a global regulatory strategy. To be effective in this role, the regulatory affairs professional must understand the international regulatory requirements the device will be subject to and ensure that the product development team considers these international requirements as they test and validate the device.
Marketing Applications
When product design is completed and a company is ready to market the device, the product development team relies on regulatory affairs to draft a successful marketing submission. In the United States, the most common type of submission is a premarket notification, also known as a “510(k),” named for Section 510(k) of the Federal Food, Drug, and Cosmetic Act, which provides the U.S. Food and Drug Administration (FDA) with the legislative authority to regulate medical devices.
To complete a successful 510(k), regulatory affairs must identify a similar device already legally marketed, known as a predicate. The submission must then demonstrate that the new device is substantially equivalent to the predicate one. If no predicate is available, regulatory affairs must submit a premarket approval application, or PMA, to demonstrate that the device is safe and effective for its intended uses.
Regulatory affairs is also responsible for reviewing all product labeling associated with the product to ensure all claims are consistent with the approved intended use. Marketing claims are key for device sales teams to differentiate your company’s product from that of a competitor. But any claims that speak to a use that has not been cleared or approved by the FDA puts the company at risk of receiving a Warning Letter for misbranding. As a result, medical device companies rely on their regulatory affairs teams to ensure all materials associated with the device are appropriate.
Postmarket Surveillance
Medical device companies also rely on their regulatory affairs team after a device is cleared or approved. In the postmarket setting, the regulatory function is involved in device surveillance, ensuring that any adverse events or malfunctions are appropriately reported to FDA.
Postmarket surveillance reports enable companies to identify trends in complaint data and can act as a warning system when a device has an unintended effect. For instance, if a company receives several adverse event reports indicating an implanted device is causing unexpected pain weeks after surgery, it’s possible that there’s an issue with the device that is causing the pain. Regulatory staff review postmarket surveillance reports and work with the FDA to initiate voluntary recalls in these situations to prevent unintended harm to patients.
Medical Devices and International Regulatory Affairs
While interactions with the FDA are key in the United States, regulatory affairs must also work with international health authorities and regulators in each country their company decides to market its device. In some countries with less mature medical device regulations, marketing clearance or approval by the FDA can be relied upon to obtain clearance or approval.
Many countries throughout the world have their own medical device regulations, however, and regulatory affairs is required to understand the differences in global regulatory requirements to ensure that new products can be marketed. The global regulatory landscape is always changing, so successful regulatory affairs teams follow global trends and are aware of new regulations or programs.
Following international harmonization organizations, such as the World Health Organization or International Medical Device Regulators Forum , can help to monitor these global trends and understand how different health authorities are dealing with an issue. Additionally, participating in industry trade associations can allow regulatory professionals to connect with one another and work collectively as an industry to identify and shape policy trends.
Regulatory affairs professionals play a key role in all phases of the product lifecycle. While each product they support provides essential knowledge and experience that can be leveraged in the future, every new product also represents a new challenge—making regulatory affairs a fulfilling career.
To learn more about advancing your career in regulatory affairs, including the skills and education required to get ahead, download our free guide below.
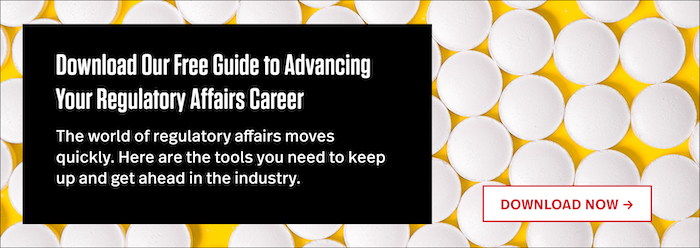
Subscribe below to receive future content from the Graduate Programs Blog.
About jeff shiffman, related articles.
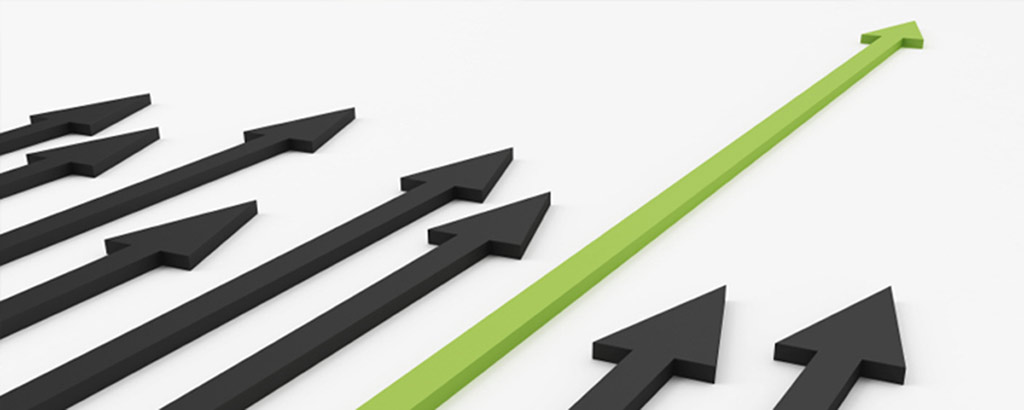
4 Ways to Stay Competitive in Regulatory Affairs
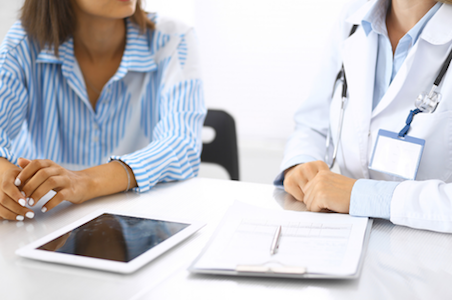
Emerging Trends in Regulatory Affairs in 2022
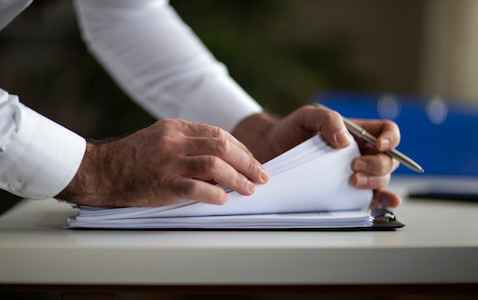
How to Stay Updated on Regulatory Changes
Stay in-demand.
64% of regulatory affairs professionals hold an advanced degree. (RAPS, 2020)
Regulatory Affairs Graduate Programs
Become qualified to manage global regulatory processes and develop cutting-edge products in healthcare and food safety.
Most Popular:
Tips for taking online classes: 8 strategies for success, public health careers: what can you do with an mph, 7 international business careers that are in high demand, edd vs. phd in education: what’s the difference, 7 must-have skills for data analysts, in-demand biotechnology careers shaping our future, the benefits of online learning: 8 advantages of online degrees, how to write a statement of purpose for graduate school, the best of our graduate blog—right to your inbox.
Stay up to date on our latest posts and university events. Plus receive relevant career tips and grad school advice.
By providing us with your email, you agree to the terms of our Privacy Policy and Terms of Service.
Keep Reading:
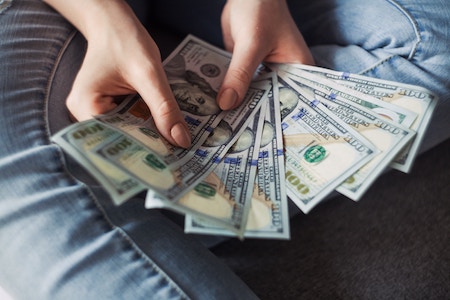
The 8 Highest-Paying Master’s Degrees in 2024
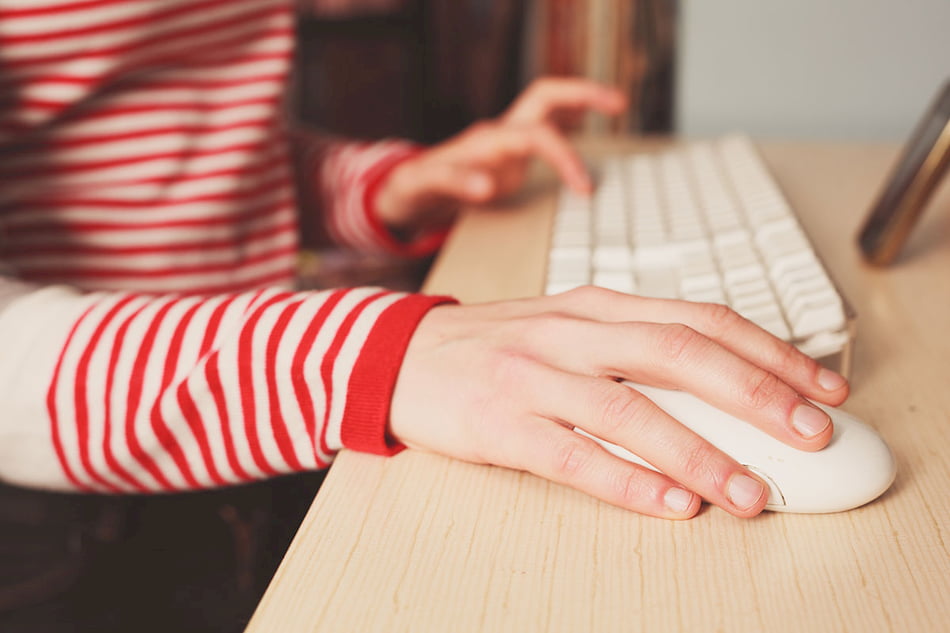
Graduate School Application Tips & Advice
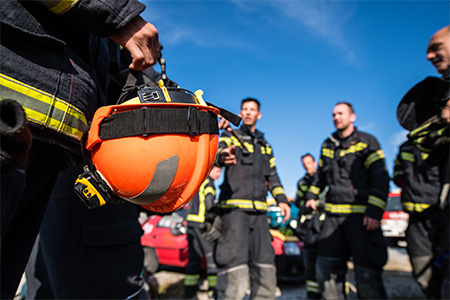
How To Get a Job in Emergency Management
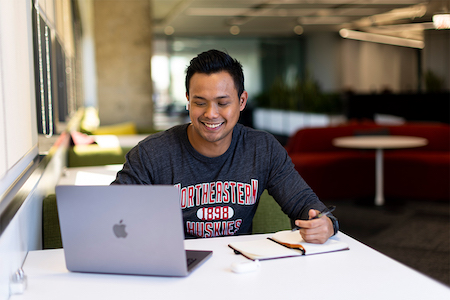
Join Us at Northeastern’s Virtual Graduate Open House | March 5–7, 2024

An official website of the United States government
The .gov means it’s official. Federal government websites often end in .gov or .mil. Before sharing sensitive information, make sure you’re on a federal government site.
The site is secure. The https:// ensures that you are connecting to the official website and that any information you provide is encrypted and transmitted securely.
- Publications
- Account settings
Preview improvements coming to the PMC website in October 2024. Learn More or Try it out now .
- Advanced Search
- Journal List
- JMIR Med Educ
- PMC10799279
Challenges for Medical Students in Applying Ethical Principles to Allocate Life-Saving Medical Devices During the COVID-19 Pandemic: Content Analysis
Hsing-yen hsieh.
1 School of Medicine for International Students, College of Medicine, I-Shou University, Kaohsiung, Taiwan
Chyi-her Lin
2 Department of Pediatrics, E-Da Hospital, Kaohsiung, Taiwan
3 Holistic Medicine, Department of Family and Community Medicine, E-Da Hospital, Kaohsiung, Taiwan
4 Data Science Degree Program, National Taiwan University and Academia Sinica, Taipei, Taiwan
5 Division of Family Medicine, Taipei Tzu Chi Hospital, Buddhist Tzu Chi Medical Foundation, and School of Medicine, Tzu Chi University, Hualien, Taiwan
Guan-chun Lin
Jhen-yu lin, clydie aldana, associated data.
Global distribution of international medical students.
The emergence of the COVID-19 pandemic has posed a significant ethical dilemma in the allocation of scarce, life-saving medical equipment to critically ill patients. It remains uncertain whether medical students are equipped to navigate this complex ethical process.
This study aimed to assess the ability and confidence of medical students to apply principles of medical ethics in allocating critical medical devices through the scenario of virtual patients.
The study recruited third- and fourth-year medical students during clinical rotation. We facilitated interactions between medical students and virtual patients experiencing respiratory failure due to COVID-19 infection. We assessed the students’ ability to ethically allocate life-saving resources. Subsequently, we analyzed their written reports using thematic analysis to identify the ethical principles guiding their decision-making.
We enrolled a cohort of 67 out of 71 medical students with a mean age of 34 (SD 4.7) years, 60% (n=40) of whom were female students. The principle of justice was cited by 73% (n=49) of students while analyzing this scenario. A majority of them expressed hesitancy in determining which patient should receive life-saving resources, with 46% (n=31) citing the principle of nonmaleficence, 31% (n=21) advocating for a first-come-first-served approach, and 25% (n=17) emphasizing respect for patient autonomy as key influencers in their decisions. Notably, medical students exhibited a lack of confidence in making ethical decisions concerning the distribution of medical resources. A minority, comprising 12% (n=8), proposed the exploration of legal alternatives, while 4% (n=3) suggested medical guidelines and collective decision-making as potential substitutes for individual ethical choices to alleviate the stress associated with personal decision-making.
Conclusions
This study highlights the importance of improving ethical reasoning under time constraints using virtual platforms. More than 70% of medical students identified justice as the predominant principle in allocating limited medical resources to critically ill patients. However, they exhibited a lack of confidence in making ethical determinations and leaned toward principles such as nonmaleficence, patient autonomy, adherence to legal and medical standards, and collective decision-making to mitigate the pressure associated with such decisions.
Introduction
The COVID-19 pandemic has caused millions of deaths and countless hospitalizations worldwide owing to critical conditions caused by the virus [ 1 ]. This has raised the ethical dilemma of allocating scarce life-saving devices to critically ill patients [ 2 - 5 ].
Physicians often make clinical decisions based on scientific evidence to avoid moral distress [ 3 , 6 , 7 ]. However, clinical decisions may have to be made under time constraints. Preparing physicians to apply appropriate ethical principles, have self-confidence in making choices, and prevent moral trauma has become essential during the pandemic [ 8 ].
The principles of autonomy, justice, beneficence, and nonmaleficence commonly serve as guiding references for allocating scarce medical resources [ 9 ]. However, these principles have multiple interpretations when facing limited resources and can be based on utilitarianism, egalitarianism, or deontology [ 10 ]. Utilitarianism believes that the primary obligation is not to treat people equally, but to maximize the greatest amount of happiness for the greatest number of people; the best actions would be based on what brings the best benefit. By contrast, egalitarianism upholds the rights and interests of individuals, which should be equally protected [ 10 ]. Deontology judges the morality of choices by its conformity with a moral norm [ 11 ], regardless of its consequences. Persad et al [ 12 ] present a comprehensive framework for the allocation of scarce medical resources grounded in the core principles of autonomy, justice, beneficence, and nonmaleficence. Their framework encompasses 4 distinct ethical value categories, including equal treatment, prioritization of the most vulnerable, maximizing overall benefits, and recognition of social usefulness. Within each category, 2 competing ethical principles emerge, yielding a total of 8 subprinciples that provide detailed guidance aligned with the overarching ethical values [ 12 ]. The core values or principles that medical students prefer or overlook when facing ethical dilemmas are unclear and require further study.
The School of Medicine for International Students at I-Shou University has a 4-year Doctor of Medicine program that collaborates with the Ministry of Foreign Affairs and enrolls college graduates from countries with official diplomatic ties to Taiwan. Due to the limited medical resources in such students’ home countries, they may face the challenge of a shortage of life-saving medical facilities in clinical practice. Therefore, equipping them with the knowledge and skills to allocate life-saving medical devices to critically ill patients, based on reasonable principles of medical ethics, is crucial. The use of virtual patients for teaching medical humanities may strengthen the effectiveness of medical ethics education [ 13 , 14 ]. Considering the challenges imposed by the COVID-19 pandemic, this solution aims to offer a secure and personalized training environment, transcending the boundaries of time and space. By doing so, students can become fully engrossed in virtual scenarios, enriching their learning experiences.
The objective of this study was to assess the ability and confidence of medical students to apply principles of medical ethics in allocating critical medical devices through the scenario of virtual patients.
Study Design
We designed a virtual scenario and asked medical students to allocate lifesaving medical devices to only 1 patient. In this scenario, a 62-year-old COVID-19-infected patient with respiratory failure was admitted to the intensive care unit. Medical students were instructed to interview a virtual patient and review the patient’s laboratory and imaging findings. They then were asked to make clinical diagnoses and adopt appropriate ethical principles to determine whether to remove the extracorporeal membrane oxygenation (ECMO) device from an 80-year-old patient currently using it and reallocate it to the new younger patient. After making their decision, the students were requested to write a short essay addressing the ethical conflicts they encountered in making the choice.
Ethical Considerations
We explained the rationale for this qualitative study and recruited third- and fourth-year medical students from the School of Medicine for International Students Program when they undertook clinical rotation at the hospital. All participants completed the virtual clinical scenarios within 4 hours in May 2021, during the COVID-19 pandemic in Taiwan, after signing an informed consent form. This study was approved by the E-Da Hospital Institutional Review Board (no. EMRP05109N and EMRP04111N), and the data were not identifiable. The teaching and evaluation of students were not affected by whether they participated in the research.
Case Scenario
Leona is a 62-year-old retired woman. She had been well without any underlying disease until recently being diagnosed with COVID-19 pneumonitis. Her lung condition continuously deteriorated, and ECMO was the last resort to support her tissue oxygenation. However, the only available ECMO machine was currently being used by an 80-year-old patient with multiple chronic illnesses who remained unstable after receiving ECMO treatment, with minimal chances of recovery.
The students were given the above scenario to assess and answer relevant questions. One of the questions was “Will you continue to let the 80-year-old patient use the ECMO, or let Leona use the ECMO instead? Please explain your decision and your reasons to support it.”
The medical students could use the 4 principles of medical ethics or base their responses on their individual analytical perspectives and reasoning for the allocation of limited medical resources.
Data Analysis
Age (>25 vs ≤25 years) and sex (male vs female) served as basic demographic variables, with the age of 25 years as a threshold of maturity. Grade (third vs fourth year) represented differences in clinical exposure experiences [ 15 ]. Textual content analysis was performed by 2 of the authors to search for keywords and summarize the students’ responses independently. The keywords were encoded and categorized for both quantitative and qualitative analyses. We used the principles of summative content analysis, which combines the quantitative counting of specific content or words or terms with latent content analysis to identify and categorize their meanings. In brief, we created a new coding category for any newly introduced terms in the assignment, and then assessed conceptual similarities to determine whether to further organize these codes into additional categories with appropriate names.
The qualitative analysis consisted of the following steps:
- The coding items included the final decision of the students (for whom to use), which core medical ethical principles were applied with various degrees in their choices, and whether viewpoints other than ethics, such as medical guidelines or legislation, were mentioned.
- The reasons for the students’ final decisions were classified according to the patient they selected, either the 62-year-old younger patient or the 80-year-old patient with multiple comorbidities. Our analysis focused on encoding the ethical justifications provided by the medical students to support their final decisions. We omitted considerations related to their alternative choices during the decision-making process.
- The classification of reasoning for those who made a decision was primarily based on the students’ understanding and interpretations in their essays, which Persad et al [ 12 ] mentioned were equality, vulnerability, maximizing the quality of life, and contribution to society. The original resource allocation principles were designed for the distribution of medical supplies among a group of individuals. However, the present case pertains to the treatment decision for an individual patient, further complicated by the fact that one patient had already been put on a ventilator. By contextualizing the principles within the framework of the present case, we eliminated the applicability of 4 subprinciples: lottery, saving the most lives, reciprocity, and giving priority to the worst off (ie, sickest first).
- If students displayed reluctance in making a choice, we also coded their explanations for the perception that ethical decision-making might not be suitable, categorizing these explanations as “undetermined” or “both unqualified.”
- The main reasons for the students’ final decisions were classified into medical, legal, and ethical perspectives.
- The coding process was independently judged by 2 researchers with expertise in qualitative research. Any inconsistencies in coding were resolved by reviewing the classification descriptions to refine the precision of category definitions and revisiting the context to ensure accurate coding.
Student Demographics
From 2021 to 2022, a total of 71 international third- and fourth-year clinical medical students who were facing the COVID-19 pandemic most significantly were enrolled. Of these, 67 students (33 third-year and 34 fourth-year students) from 12 countries participated in the study. Because 4 fourth-year medical students did not participate, the response rate was 94%. Overall, 40 (60%) participants were female and 61 (91%) were older than 25 years. Most medical students were from the Kingdom of Eswatini, accounting for 48% (n=32) of the total group ( Table 1 and Multimedia Appendix 1 ).
Basic information of the students.
Choosing the Best Candidate for ECMO Allocation
Of the 67 participating students, age group (<25 vs ≥25 years old), sex (male vs female), and seniority year (third vs fourth year) did not affect patient selection preferences, and a larger proportion of students from Eswatini (21/32, 66%) selected the 80-year-old patient for ECMO compared to the rest of the students (39/67, 58%). The majority of students decided to continue treating the 80-year-old patient with ECMO ( Table 2 ).
Choosing the most suitable patient for extracorporeal membrane oxygenation treatment.
Additionally, 5 (8%) students argued that the medical information provided was not sufficient to make decisions that were highly dependent on factors such as the patient’s condition, the course of the disease, and legal requirements. One student (1%) suggested that, in accordance with medical guidelines, neither patient met the conditions to be a candidate for ECMO. A possible reason for them to abstain from decision-making could be the pressure they experienced while facing an ethical dilemma. As one student (no. 16) stated:
Doctors should not take the treatment away of one person and give it to another, regardless of the odds of survival rate of these two patients, because it means that we are taking the role of God, deciding who lives and who dies.
Another student (no. 20) stated:
I don't believe I have the right to decide who is more deserving or who needs this equipment more.
Students’ Perspective of Allocating Limited Resources
Building upon the framework proposed by Persad et al [ 12 ], this study identified 4 coding categories after excluding subprinciples that were deemed inapplicable to the current case. In accordance with the students’ final decisions regarding the most suitable recipient for ECMO, we categorized the reasons endorsed by the students ( Table 3 ). The primary justifications for selecting an 80-year-old patient included nonmaleficence (n=31, 46%), first-come-first-served (n=21, 31%), and patient autonomy (n=17, 25%). Students grounded their decisions in 3 of the 4 ethical principles, arguing that in this particular scenario, those advocating for the principle of nonmaleficence contended that physicians lacked the authority to withdraw a life-saving device in active use. “First-come-first-served” represents 1 of the 4 interpretive angles of the justice principle from Persad’s framework. Students believed that the life of each patient held equal value, and those who received treatment first should be allowed to continue treatment. Students who mentioned patient autonomy were particularly concerned about the absence of informed consent and its potential legal implications for health care providers.
Multiple-choice analysis of the reasoning for case selection among students.
a ECMO: extracorporeal membrane oxygenation.
The reasons for selecting the 62-year-old patient primarily revolved around the principle of justice. The utilitarian principle of maximum benefit was the most popular: 31% (n=21) of students mentioned that medical resources should be reserved for patients who can survive the longest and have the best quality of life. When comparing who had better survival probabilities, some students suggested that medical guidelines should serve as the basis for the final decision. Overall, 10% (n=7) of students made decisions depending on who had contributed more to society as a whole, and 4% (n=3) prioritized the disadvantaged, where the disadvantaged can be interpreted as the younger patient.
Students who expressed an “undetermined” stance believed that decision-making authority should be entrusted to guidelines, which could be either principles collectively established by physicians within the hospital (n=4, 6%), hospital policies (n=4, 6%), local laws (n=4, 6%), or decisions made by the hospital’s ethics committee (n=3, 4%). Alternatively, some advocated for decisions to be made collectively by physicians within the hospital (n=1, 1%), by the patients’ families (n=1, 1%), or based on other information relevant to the patient’s condition (n=1, 1%). One student expressed a “both unqualified” position and approached the issue from a medical rather than an ethical perspective. The student asserted that, based on the guidelines, neither of the 2 patients met the criteria for usage.
Adequacy of Using Medical Ethical Principles
In total, 73% (n=49) of students cited the principle of justice while analyzing this case. When ethical principles were in conflict, the principle of justice was most commonly cited. The frequencies of ethical principles considered by medical students in making final decisions (coding as simple choice) were as follows: 48% (n=32) used the principle of justice, 25% (n=18) used the principle of nonmaleficence, 12% (n=8) used the principle of patient autonomy, and 9% (n=6) were unable to provide a definitive response.
Confidence in Ethical Decision-Making
Overall, 75% (n=50) of the participants analyzed the case from other perspectives, such as medicine and law, and 25% (n=18) made their final decision based on the principles mentioned in the clinical guidelines. These students were more inclined toward the scientific mode of thinking, believing that evidence-based medicine is objective and may provide clear standards that can give them a sense of security. Students no. 23 and 31, respectively, indicated the following:
I can respond to this situation based on scientific evidence.
A comprehensive assessment of the pathology of the patient’s current condition and the state of illness is a major consideration in decision-making.
For 12% (n=8) of the medical students, their final decisions were made from a legal perspective; that is, they stated that the decision should be made in accordance with the law of the state. They emphasized that physicians should protect themselves from being sued and provide decision-making authority to the patient or family. The patients or their family members should sign the emergency consent form, allowing the patient or family to participate in decision-making. As stated by student no. 40:
Medical care providers must consider medical laws, including those for removing the machine from the patient and withholding services from patients.
Additionally, 6% (n=4) of the medical students believed that medical institutions should provide clear guidelines or set up ethics committees to make collective decisions, thus preventing individual doctors from facing the pressure of decision-making. Student no. 18 stated:
I will follow the organization's code of ethics. The handling rules approved by a specific organization that will guide you in such situations so that you do not face a violation of the law.
Principal Findings
ECMO is recommended for severe COVID-19-related acute respiratory distress syndrome to reduce mortality [ 16 ]. Currently, there is no evidence-based ethical guidance for prioritizing ECMO when resources are limited during the COVID-19 pandemic [ 17 ]. Justice is the preferred principle in virtual settings, although students have diverse interpretations. Nearly half of the students used additional principles, such as nonmaleficence and respect for patient autonomy, to prevent further harm while making ethical decisions. Multiple perspectives were adopted by three-fourths of the students.
The context of clinical situations is important for making clinical decisions based on ethical dilemmas [ 18 ]. The use of virtual patients for medical education may strengthen the effectiveness of medical ethics education [ 13 , 14 ]. Using virtual patients for clinical decision-making training among international medical students offers several advantages [ 19 - 21 ]. It provides a safe training environment amidst the COVID-19 pandemic and allows for diverse case presentations from multiple countries and cultures [ 22 ]. The application of virtual care has flourished internationally during the post-COVID era. The Cleveland Medical Center in the United States has also explored the integration of remote and virtual health care. Medical institutions in the southern United States have proved that virtual diagnosis and treatment can alleviate caregiver burden and promote patient care [ 23 ]. Our study has provided evidence that combining virtual training with ethical reasoning in solving ethical dilemmas may present a safe environment for learning clinical decision-making and offer opportunities for improvement.
Students were asked to think about and answer questions according to the situation of the virtual patient. More than half of the students chose the oldest or the sickest patient to be the best candidate. The clinical scenario that was tested involved ex-post triage, which entails discontinuing ongoing treatment in favor of a newly arrived patient. Particularly in the context of a pandemic with limited resources (eg, ventilators), the primary objective is to maximize overall benefits for all individuals. While challenging, medical physicians may need to make the difficult decision of reallocating life-saving facilities from the most critically ill patients to those who have a higher probability of survival [ 5 ]. During a pandemic, rationing may require the withdrawal of care in order to provide ventilators to patients who are given higher priority, a reason foreign to many front-line clinicians [ 24 ]. Sharing and leveraging the diverse responses of medical students themselves can serve as a valuable reference for fostering innovative approaches in medical ethics education and facilitating ethical deliberation on challenging medical issues.
Medical students must define problems, identify potential solutions, and also inform patients about the current treatment options. The students’ understanding of patient autonomy and informed consent was superficial and formalistic; they were more concerned about obtaining consent or documents to avoid legal proceedings. Recent discussions on the principles of patient autonomy have concluded that superficial autonomy cannot guarantee patient autonomy [ 25 - 27 ]. Moreover, physicians should make more efforts to meet the best interests of patients [ 28 , 29 ]. Considering students’ diverse backgrounds, it is important to take into account their various learning styles to enhance and personalize educational materials [ 30 ].
The inability to establish a definitive ethical guideline capable of resolving issues stemming from the scarcity of medical resources underscores the complexity of the situation. Furthermore, factors such as patients possessing varying medical needs, financial capabilities to cover medical expenses, and the policies of health care institutions can all impact the ethical judgments of students [ 31 , 32 ]. Therefore, teachers can take the opportunity to emphasize to students that the premise of patient autonomy and informed consent is to uphold the patient’s right to live, and promoting the well-being of the patient is the core value of the principle of patient autonomy. To ensure the patient’s autonomy is respected, physicians should make decisions that benefit the patient’s overall health and care.
Students were unfamiliar with philosophical and ethical reasoning and were under pressure to make ethical decisions about allocating life-saving medical modalities. They tended to analyze ethical issues from both medical and legal perspectives [ 33 , 34 ]. Most medical students relied on objective medical guidelines, legal documents, or hospital management systems to help them make decisions while lacking life-saving medical modalities. Experts might erroneously assume that by dutifully adhering to the code’s regulations they fulfill all pertinent ethical obligations. Similarly, many people hold the belief that by fulfilling all applicable legal prerequisites, they have fulfilled their moral duties. It is important to note that what may be deemed ethically correct does not always find support within the confines of the law. Legal education places emphasis on the introduction of statutes and their applicability, while ethical education delves into the reasoning process underlying diverse ethical decisions. Within medical ethics education, an exploration of students’ abilities to discern the implications of various ethical decisions and make informed value judgments is paramount [ 35 ]. Some students believe that developing medical guidelines can serve as a substitute for individual ethical decision-making. Use of the specification method to solve ethical dilemma questions has limitations. If a specification eliminates contingent conflict, it may be arbitrary, lack impartiality, or fail for other reasons. We cannot avoid judgements that balance different principles or rules in the very act of specifying them. It also seems pointless or unduly complicated to engage in specification in many circumstances [ 35 ].
To foster the development of medical students’ ethical thinking, it becomes crucial to provide them with opportunities to analyze cases using established ethical frameworks with proper guidance [ 5 ]. Furthermore, facilitating the sharing of diverse perspectives on case analysis can also prove valuable in nurturing community-specific morality, which draws its foundations from culture, religion, and institutional systems [ 35 ]. Based on our study, we proposed that the necessity of strengthening medical ethics education stems from the following: acknowledging physicians’ needs for independent ethical decisions during a pandemic, recognizing the irreplaceability of clinical ethical judgment over legal rules and medical guidelines, elevating students’ ethical reasoning abilities, and elucidating the core value and application scope of patient autonomy.
This study explored the current status of critical ethical decision-making from the diverse perspectives of international medical students and provided information using a virtual patient scenario. Heist et al [ 36 ], using case summaries, found that 5 sessions of virtual patient case scenarios significantly improved students’ clinical reasoning abilities. In light of the rapid advancement of virtual medical education platforms amidst the COVID-19 pandemic, it is suggested that medical schools proactively integrate a series of diverse virtual patient ethics decision-making exercises. This strategic inclusion aims to foster robust and well-rounded ethical education training for medical students, equipping them with the necessary skills to navigate complex ethical dilemmas in their future medical practice.
Through incorporating the survey in the formal class activity, we received a robust 94% response rate from a diverse group of medical students [ 37 ]. However, this study has some limitations. First, the interface and language processing technique of the virtual system could be more user-friendly in mimicking the true clinical interaction with patients. The responses of virtual patients were based on a predetermined script derived from a limited database design, making it difficult to respond to students’ more in-depth or spontaneous questions. Second, owing to the limited number of participants (n=67) and the fixed setting of a single virtual patient, students’ responses may not have been extrapolated. If the current medical resources and institutional policy differ, students might make various decisions.
This study addressed the need for practical clinical ethics training in medical education by using virtual patients to offer students simulated scenarios for cultivating decision-making experiences. It compiled diverse perspectives from students of various cultural backgrounds, enhancing their capacity for comprehensive ethical considerations. The research suggests a more effective curriculum development approach by combining individual case studies with a collective analysis of answers. As future physicians, these students will benefit from this training when making time-sensitive ethical decisions based on all stakeholders’ viewpoints. This study also identifies a lack of student confidence in making ethical decisions related to patients’ lives. It highlights the need to foster the independent ethical decision-making competency of medical students.
Acknowledgments
We thank the School for International Medical Students, College of Medicine of I-Shou University for offering the teaching material and facilities; the library of the E-Da Hospital for research resources and space; and the National Science and Technology Council for their support. This project was funded by the National Science and Technology Council, Taiwan (grants MOST-109-2511-H-650-002-MY2 and MOST 111-2410-H-650-002).
Abbreviations
Multimedia appendix 1.
Authors' Contributions: H-YH contributed to the conception of this work, data analysis and interpretation, and writing of manuscript. RYH contributed to the conception of work, data acquisition, writing of the manuscript. G-CL contributed to data analysis and interpretation. J-YL and CA contributed to the substantial revision of the manuscript with English editing. C-HL contributed to the conception of this work, oversaw the quality, and contributed to substantial revisions. The authors have read and approved the final manuscript.
Conflicts of Interest: None declared.
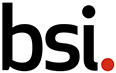
Making excellence a habit
- Verify a certificate
Buy standards

Popular searches
- ISO 13485 Quality Management for Medical Devices
- ISO 14971 Risk Management for Medical Devices
- ISO 27001 Information Security
- ISO 45001 Occupational Health and Safety Management
- Training courses
Suggestions
- Medical Devices
- Whitepapers and Articles

Whitepapers and articles
Download the latest medical device whitepapers and articles below to help you perform better, reduce risk and make excellence a habit in your organization.
Recent whitepapers
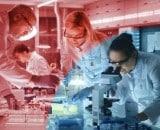
Using standards to demonstrate conformity
The application of standards in the medical devices sector has undoubtedly been accelerated by their use to support regulation by providing a voluntary means to demonstrate conformity with regulatory requirements.
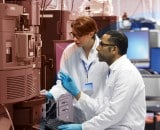
Medical device clinical investigations — What’s new under the MDR?
This paper was first published by BSI in 2018 and has been revised in light of the publication of BS EN ISO 14155:2020. The paper discusses important requirements for pre-market and post-market clinical investigations under the European Medical Device Regulation (2017/745) (MDR), relevant European guidance documents and BS EN ISO 14155:2020, and how this updated standard can help in meeting MDR requirements.
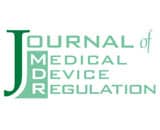
A notified body’s perspective on the clinical evaluation requirements under the Medical Device Regulation
Understanding of the clinical evaluation process for medical devices against the requirements of the MDR, relevant Medical Device Coordination Group guidance documents is critical for all manufacturers.
Hear from BSI’s Richard Holborow, Head of Clinical Compliance in his recent article in the Journal of Medical Device Regulation on the requirements for clinical evaluation under the MDR from a Notified Body’s perspective and how to meet those requirements.
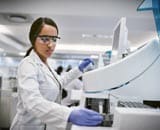
EU MDR and IVDR: unique device identification
This white paper provides an overview of the EU UDI system, its requirements and the status of EUDAMED, along with some practical recommendations for manufacturers to support their UDI system compliance efforts.
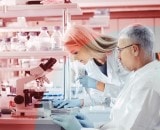
Phthalates and endocrine disruptors
Phthalates are plasticizers that impart flexibility to plastic products and can leach into their surroundings. Many phthalates have the potential to cause hormonal disruption. This white paper summarizes the evaluation of phthalates and endocrine-disrupting (ED) substances in medical devices (MDs).
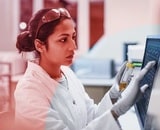
The convergence of the pharmaceutical and medical devices industries
Find out more about the different categories of combination products within the EU along with the regulatory pathways designed to ensure they are safe and perform as intended.
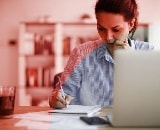
Person responsible for regulatory compliance (PRRC) - MDR/IVDR Article 15
With the MDR and IVDR, European regulators aim to ensure companies have a regulatory expert – a Person Responsible for Regulatory Compliance (PRRC) – at their disposal, to ensure that the company is meeting certain specific EU requirements.
View all whitepapers Click on the titles below to read more about each whitepaper.
Authorized representatives under the mdr > learn about the background to the new eu rules regarding authorized representatives and the actions your organization may need to take as a result of them with our dedicated guide., classification changes under the mdr > download our guide to the risk classification rules under the mdr and gain expert insight into the background to the new eu rules regarding risk classification and the actions your organization may need to take as a result of them., comparison of the articles of the european mdd and the mdr > this guide presents a summary of the provisions of some of the articles of the mdd and mdr together with commentary providing discussion and highlighting the key differences., cybersecurity of medical devices > this paper considers the cybersecurity challenges facing the healthcare sector arising from the convergence of technology, hyper-connectivity and recent developments in regulation., digital maturity in an age of digital excitement > digital technology in the medical devices sector has the power to excite. but, it is important that a mature, critical approach is taken when evaluating new technologies. download this medical device white paper today and learn about digital maturity., effective post-market surveillance > this paper explores the current regulatory and legislative requirements with regards to post-market surveillance (pms), in the wake of recent health scares concerning implants., engaging stakeholders in the home medical device market > this paper considers the implications for manufacturers when medical devices designed for trained professionals move to community use and looks at essential issues for designing devices specifically for this growing market., general safety and performance requirements (annex i) > this paper provides comparison of the safety and performance requirements (sprs) of the new medical devices regulation (mdr) and the essential requirements (ers) of the medical devices and active implantable devices directives that they replace., importers, distributors and assemblers > download our guide and gain expert insight into the background to the rules regarding importers and distributors under the mdr/ivdr and the actions your organization may need to take as a result., iso 13485 - the proposed changes and what they mean for you > this paper introduces the proposed updates to iso 13485, the quality management system standard that complements product specific directives., nanomaterials > this white paper discusses the advent and use of nanomaterials in medical devices and explores the regulatory requirements for devices containing nanomaterials., negotiating the innovation and regulatory conundrum > this paper raises important questions about the attitude to creative product development, illustrating the need for a closely documented approach throughout the process., planning for implementation of the european union medical devices regulations > this paper discusses decisions that need to be made by affected organizations and includes questions to ask about your organization’s preparedness in order to comply with the new requirements., post-market surveillance under the mdr/ivdr > gain expert insight into the background to the new eu rules regarding post-market surveillance (pms) and the actions your organization may need to take as a result of them., recent advancements in ai – implications for medical device technology and certification > the purpose of this white paper is to provide a very brief overview of where ai is being used in healthcare, and why it might be increasingly seen in medical devices., responsibilities for medical device vigilance reporting > this paper outlines the requirements specific to incident reporting, vigilance, mandatory problem reporting, medical device reports and adverse event reporting to support those working with these aspects within the mdsap program., risk management for medical devices and the new iso 14971 > this whitepaper seeks to provide a brief overview of the development of risk management from elementary risk awareness in the early days to the structured stepwise process of planning, assessment, control and monitoring that we have today., technical documentation and medical device regulation > this whitepaper reviews the regulatory requirements for technical documentation under the new mdr, with focus on conformity assessment routes and post-market surveillance., the differences and similarities between iso 9001:2015 and iso 13485:2016 > quality management professionals need to know the differences and similarities in these two revised standards in order to react to the revisions and mitigate any concerns the revisions may create., the european medical devices regulations > this paper addresses a number of areas, including pms as an element of the management of clinical evidence throughout the device lifecycle, the pms system, the pms plan; preparation of a summary report of pms information, complaint handling and more., the growing role of human factors and usability engineering for medical devices > by applying knowledge of human capabilities and limitations human factors and usability engineering (hf/ue) contributes to the design of intuitive displays, controls, and other interfaces that substantially reduce the risk of user error., the impact and potential for 3d printing and bioprinting in the medical devices industry > this whitepaper seeks to review the history of 3d printing of medical devices, identify the key characteristics of successful exploitation, and to examine the scope for bioprinting processes to enhance medical devices., the post-market priority > dr. hamish forster, bsi orthopaedic and dental product expert, provides an overview of the key elements required within technical documentation to demonstrate conformity to the medical device directive 93/42/eec., the role of the person responsible for regulatory compliance > download our guide to learn more about the background to the eu rules regarding responsible persons and the actions your organization may need to take as a result of them., udi and the mdr/ivdr > download our guide and gain expert insight into the background to the new eu rules relating to unique device identification (udi) and the actions your organization may need to take as a result of them., what you need to know about the fda’s udi system final rule > taking an in-depth look to explore standardized granular identification of medical devices, associated meta-data to support various public-health initiatives and step by step guidance and advice for udi compliance..

Home › Featured news › The impact of IoT on medical equipment and healthcare
The impact of IoT on medical equipment and healthcare
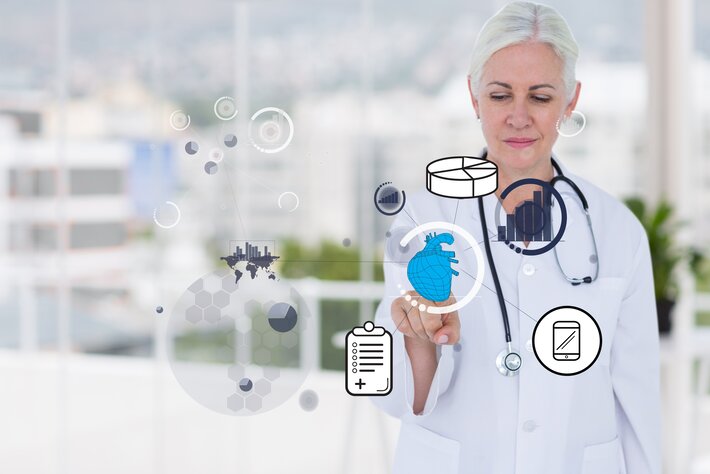
In the healthcare industry , medical equipment and medical IoT have become an important part of treatment. More and more connected devices are not only changing patient care but also improving medical intelligence. With the help of technical innovation, medical devices are not only reducing operational costs but also providing a promising path for improving health outcomes for billions around the globe.
What is IoT in healthcare?
IoT in healthcare is the network of connected medical devices and apps that collect, send and receive data. This includes devices that are worn, such as fitness trackers, and others that are complex in-hospital machines collecting data on patients’ vital signs. IoT enables these devices to communicate with one another and share this data, improving the ability of doctors, nurses or family members to deliver effective care in a timely manner.
Benefits of IoT in medical equipment
The integration of IoT in medical equipment presents numerous benefits that significantly enhance healthcare delivery and patient outcomes :
- Better patient monitoring : IoT devices provide continuous real-time monitoring of patients both in the hospital and from home. Patients can have wearable or implantable devices to measure important health markers such as blood glucose and heart rate. Alarms can be sounded to both the patient and caregivers or hospital personnel if key vital signs reach critical ranges to enable quick response and intervention.
- Better treatment results : Physicians with real-time data make better decisions. IoT devices are collecting and analysing enough health information to allow treatment to be tailored to individual patients. This can result in better outcomes.
- Improved operational efficiencies : IoT helps administrators to operate a hospital more effectively for economic and time-saving gains, for instance. Tracking of medical instruments with IoT sensors used in monitoring will reduce waste by making sure the medicine is not spoiled and reducing the chance of it being misplaced or unavailable where needed.
- Remote patient management : IoT helps to enable telehealth services, whereby patients can be taken care of in their own home, which is valuable for patients with chronic disease and for those residing in remote areas.
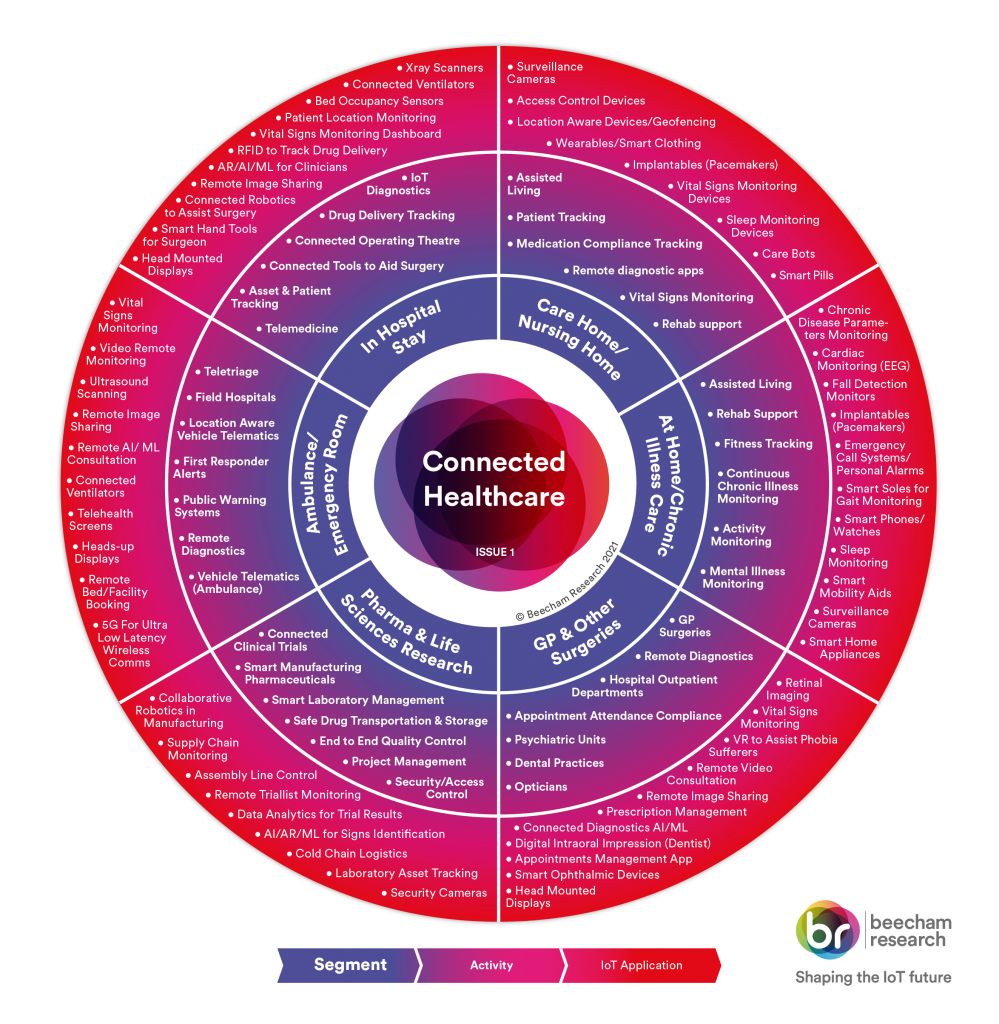
Challenges and considerations
While IoT in healthcare offers significant benefits, there are also challenges to consider:
- Data security and privacy
- Interoperability
- Regulatory compliance
Cost and data privacy are among the first things that come to mind with regard to IoT in healthcare . Data security is a major concern, as healthcare devices collect and transmit sensitive patient data that makes them prime targets for attack if the right protective measures are not taken and data protocols are not respected.
Another major issue is related to the interoperability of healthcare devices. If the devices used by healthcare providers are not built to speak the same language, they will not come together so easily. The data simply won’t flow freely, impacting not only patient care but also operational efficiency and profitability.
Then, there is regulatory compliance to think about, as healthcare IoT needs to adhere to standards to meet life-threatening situations safely and effectively. We should also consider another significant technological hurdle – not all patients are capable of using the latest technology, which potentially increases the healthcare gap.
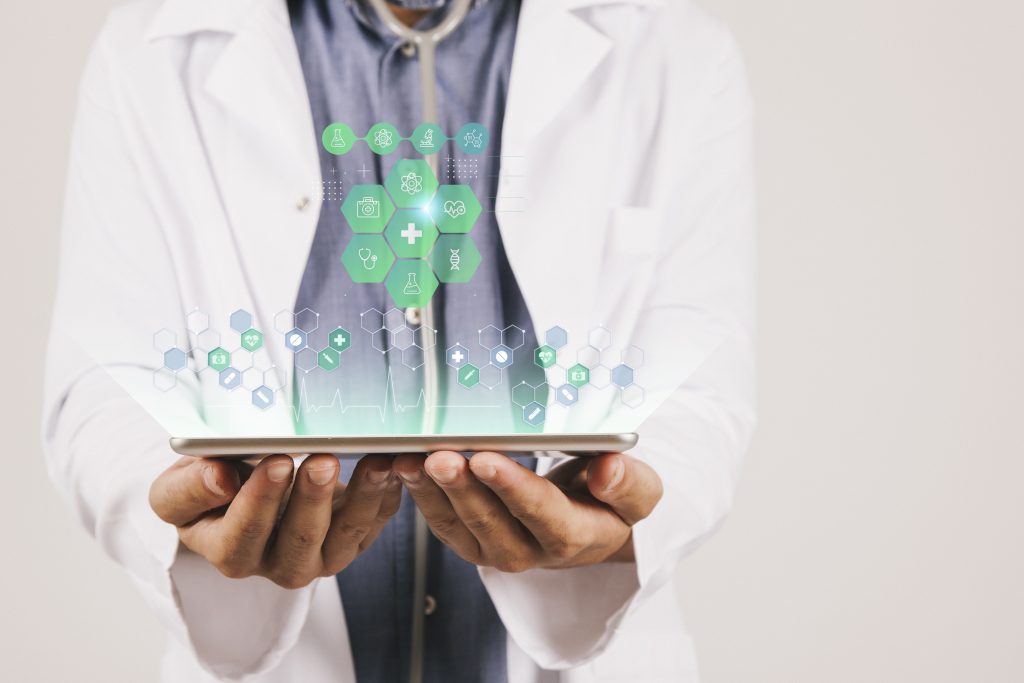
Future prospects
The future of IoT in healthcare looks promising. Advancements in AI and machine learning are set to further enhance the capabilities of IoT devices. Research from 2023 by Juniper Research predicts that 115 million patients will use remote patient monitoring by 2027. That’s 67% more than the 75 million in early 2023.
IoT is influencing all kinds of industries, so it was only a matter of time for it to also make a meaningful change within the medical field. It offers solutions that, ultimately, can save lives and improve the care of patients all over the world with the power of connectivity. This change is only going to increase. In the years to come, we can look forward to advancements and devices that will completely reshape the way hospitals and day-to-day health monitoring will work.
To ensure that this evolution is beneficial to all, it is paramount that healthcare providers and technology developers continue to work together to overcome hurdles and maximise the potential of IoT healthcare.
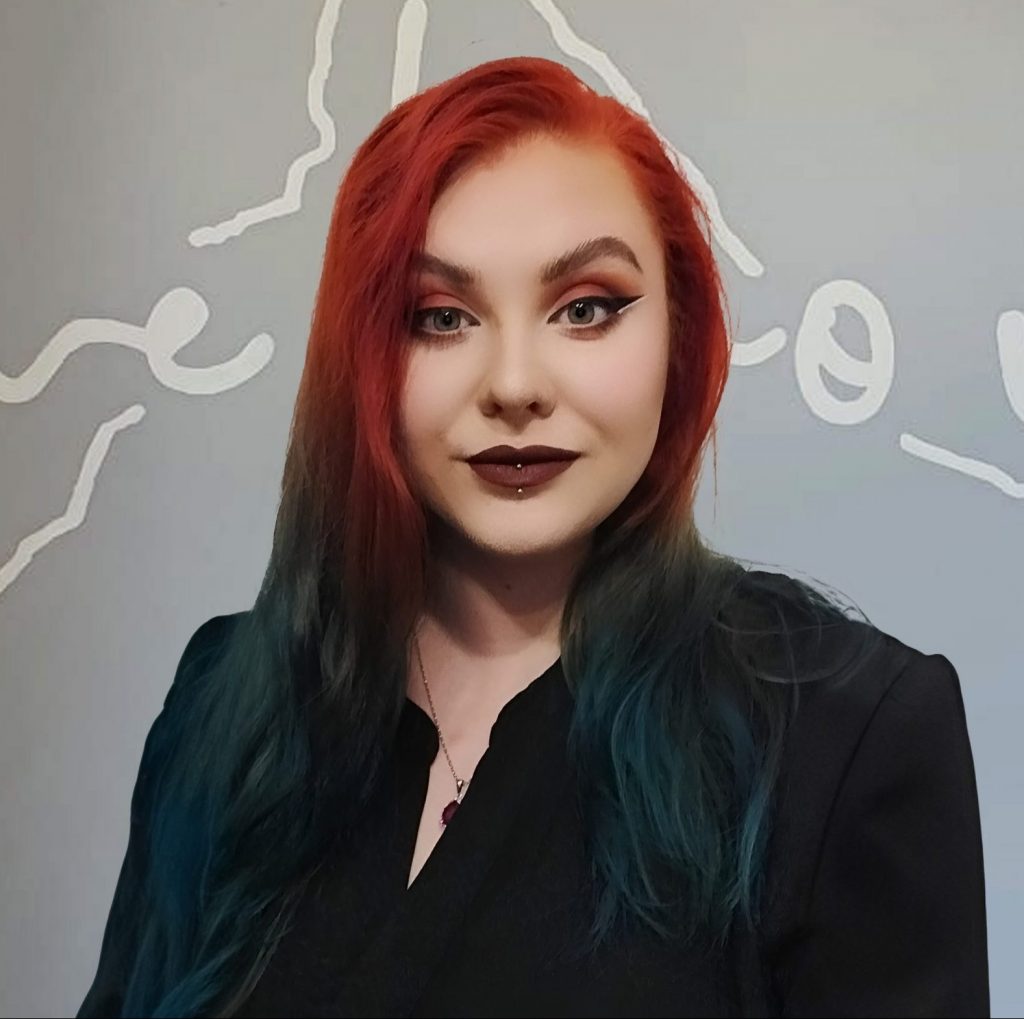
Article by Magda Dąbrowska, a technical writer at WeKnow Media
Comment on this article via X: @IoTNow_
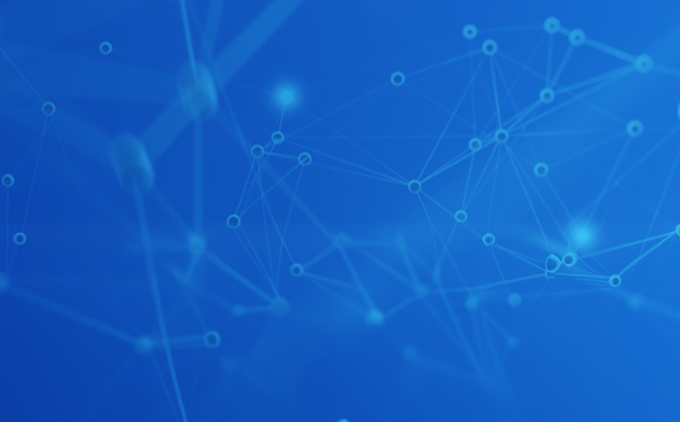
Airtel to power more than 20 million Adani smart meters
Airtel Business, the B2B arm of Bharti Airtel, has announced that it will power over 20 million smart meters for Adani Energy Solutions Limited (AESL). Airtel, through its nationwide communications
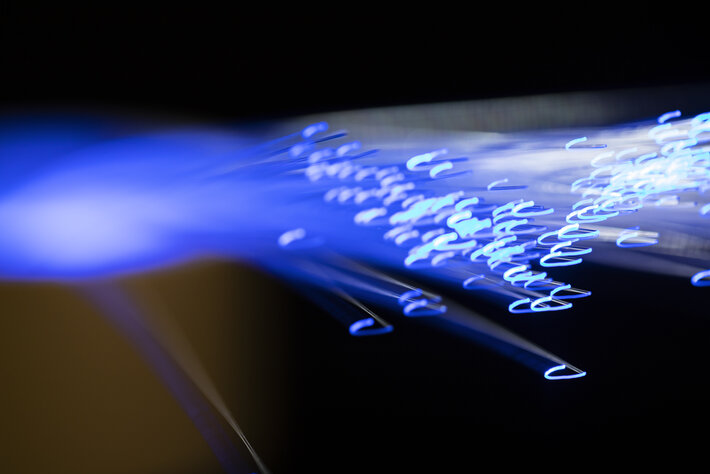
CDG and Innovation Incubator launch AI-powered telecom solutions
Communications Data Group has announced an alliance with Innovation Incubator to operate an extension of CDG’s innovation lab and develop Generative AI powered solutions aimed at transforming the subscriber and
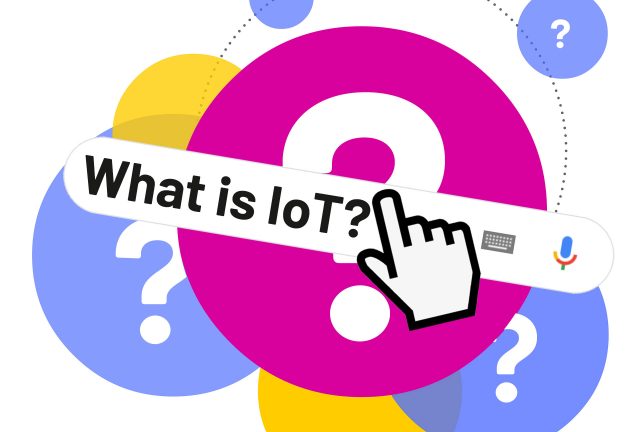
What is IoT? A Beginner’s Guide
What is IoT? IoT, or the Internet of Things, refers to the connection of everyday objects, or “things,” to the internet, allowing them to collect, transmit, and share data. This
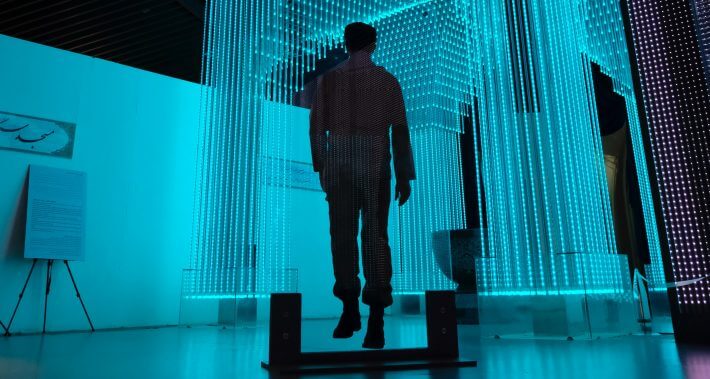
The IoT Adoption Boom – Everything You Need to Know
In an age when we seem to go through technology boom after technology boom, it’s hard to imagine one sticking out. However, IoT adoption, or the Internet of Things adoption,

9 IoT applications that will change everything
Whether you are a future-minded CEO, tech-driven CEO or IT leader, you’ve come across the term IoT before. It’s often used alongside superlatives regarding how it will revolutionize the way

Which IoT Platform 2021? IoT Now Enterprise Buyers’ Guide
There are several different parts in a complete IoT solution, all of which must work together to get the result needed, write IoT Now Enterprise Buyers’ Guide – Which IoT
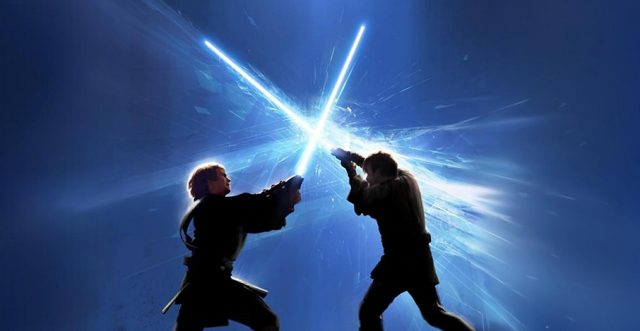
CAT-M1 vs NB-IoT – examining the real differences
As industry players look to provide the next generation of IoT connectivity, two different standards have emerged under release 13 of 3GPP – CAT-M1 and NB-IoT.
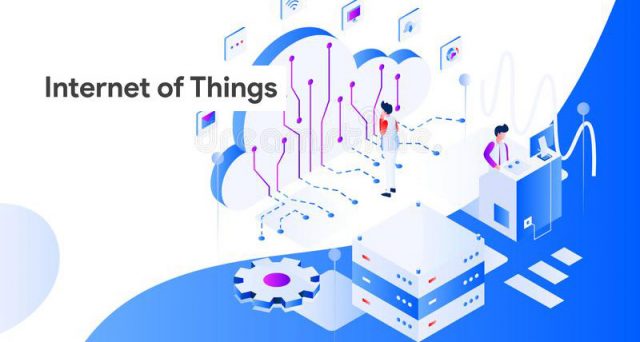
IoT and home automation: What does the future hold?
Once a dream, home automation using iot is slowly but steadily becoming a part of daily lives around the world. In fact, it is believed that the global market for
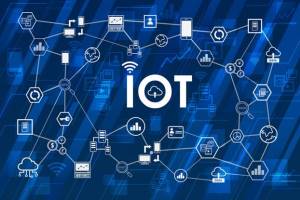
5 challenges still facing the Internet of Things
The Internet of Things (IoT) has quickly become a huge part of how people live, communicate and do business. All around the world, web-enabled devices are turning our world into
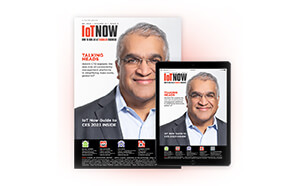
Out Now! IoT Now Magazine
Get the latest iot news to your inbox, join the iotnow online community for free, to receive:.
Exclusive offers for entry to all the IoT events that matter, round the world
Free access to a huge selection of the latest IoT analyst reports and industry whitepapers
The latest IoT news, as it breaks, to your inbox
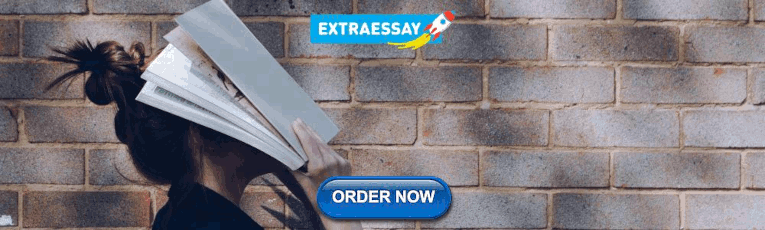
IMAGES
VIDEO
COMMENTS
Please use one of the following formats to cite this article in your essay, paper or report: APA. Aliouche, Hidaya. (2023, April 06). What are Medical Devices?.
A total of 82 relevant papers drawn from 960 papers on the subject of wearable devices in health care settings were qualitatively analyzed, and the information was synthesized. Our review shows that the wearable medical devices developed so far have been designed for use on all parts of the human body, including the head, limbs, and torso.
Digital health interventions refer to the use of digital technology and connected devices to improve health outcomes and healthcare delivery. This includes telemedicine, electronic health records, wearable devices, mobile health applications, and other forms of digital health technology. To this end, several research and developmental activities in various fields are gaining momentum. For ...
Biomedical engineers design medical devices, raising many ethical dilemmas in ordinary times, which become compelling during such a crisis. ... For this reason, a rapid search for papers regarding COVID-19 or SARS-CoV-2, and the previous epidemic/pandemics (i.e., SARS, MERS, Swine Flu) as a comparison, was performed both on OvidSP and the ...
Objective: Medical device development, from the product's conception to release to market, is very complex and relies significantly on the application of exact processes. This paper aims to provide an analysis and summary of current research in the field of medical device development methodologies, discuss its phases, and evaluate the associated legislative and risk aspects.
The U.S. Food and Drug Administration, in collaboration with device manufacturers, should establish a medical device database for physicians and other providers, including pharmacists, to use when selecting appropriate devices to prescribe or recommend. Page 178 Share Cite.
PAGES 8 WORDS 2514. Background. In June, 2014, the world's #2 maker of medical devices, Medtronic, agreed to buy Covidien, a smaller company in the same industry. The deal was valued at $42.9 billion, and it was a cash and stock deal. The deal was done at a 29% premium over Covidien's June 13, 2014 closing price.
Physicians have driven the development of numerous devices to aid their practice in the past [4,5]. Indeed, one of the defining aspects of the bond between the patient and physician, the stethoscope, was invented by a doctor. The increased use of medical devices in all aspects of medicine has presented ethical challenges.
The Journal of Medical Devices is a unique international forum for rapid publication of significant research on the development and characterization of devices including instruments aimed for biomedical applications. ... Stay up to date on the latest call for papers if you'd like to submit. Why Publish in ASME Journals?
A Critical Analysis of Ethical Issues in Medical Devices and Instruments. Introduction Modern medicine has medical devices and instruments that have improved the quality of life by mitigating disease spread, curing illnesses, and sustaining life processes from critical medical conditions. With the influx of technological innovations, medical ...
New technology in materials science, processing, and assembly, and in the health-care community combined to make the global medical device market a $200 billion market in 2008. With a growth rate of 8%, by 2012, the size grew to over $250 billion. The United States is reported to consume between 45% and 47% of the world market for medical ...
The Safe Medical Devices Act (SMDA) is federal legislation that promotes the security and safety of medical devices and products. In particular, the Act came into existence back in the 1990s owing to the need by the government to combat injuries and illnesses brought about by the consumption of wrong products (Curfman et al. 976).
medical device after being opened or used in a clinical setting (Dunn, 2002; Health, 2019). The ... essay by discussing the issue of patient consent when using reprocessed single-use devices. Collier argues that on one hand, patient consent may not be needed if hospitals have policies to
Medical equipment: A brief insight on commonly use-. in all health care settings. Bagyavathi Battini, Usha Rani Kandula, Reetha Murugesan, Siresha. Inapagolla and Gera VijithaVinni. Abstract ...
Readers are directed to additional resources such as AAMI HE75:2009, Human factors engineering - Design of medical devices, and ISO 14971 [8].. 3. Common errors while designing a medical device. A medical device design can fall away from the path and can go astray when the medical device company forgets to take into account the uncertainty factor.
The Medical Product Safety Network (MedSun) is an adverse event reporting program launched in 2002 by the U.S. Food and Drug Administration's Center for Devices and Radiological Health (CDRH); the primary goal for MedSun is to work collaboratively with the clinical community to identify, understand, and solve problems with the use of medical ...
Medical implants refer to the tissues or manufactured devices that doctors put on the surface or inside the body of a patient. Normally, doctors use medical implants to replace missing and malfunctioning body parts, deliver medication, monitor body function, and to reinforce body organs and tissues. Individuals use medical implants for health ...
Essays on Medical Device. 5 samples on this topic. Writing piles of Medical Device papers is an immanent part of modern studying, be it in high-school, college, or university. If you can do that unassisted, that's just awesome; yet, other learners might not be that skilled, as Medical Device writing can be quite challenging.
The medical device industry plays a key role in the healthcare ecosystem by providing innovative solutions that improve patient outcomes. Every company seeks to innovate, whether through iterative updates to existing technology or by developing new devices that could offer a more effective treatment to a particular disease or condition.
We designed a virtual scenario and asked medical students to allocate lifesaving medical devices to only 1 patient. In this scenario, a 62-year-old COVID-19-infected patient with respiratory failure was admitted to the intensive care unit. ... After making their decision, the students were requested to write a short essay addressing the ethical ...
The European Union (EU) Medical Devices Regulation (EU 2017/745) (MDR) and the In Vitro Diagnostic Medical Devices Regulation (EU 2017/746) (IVDR), now apply. Important terms used in the regulations are 'entry into force' and 'date of application'. The publication of the text in the Official Journal of the European Union was on April 5 ...
In the healthcare industry, medical equipment and medical IoT have become an important part of treatment.More and more connected devices are not only changing patient care but also improving medical intelligence. With the help of technical innovation, medical devices are not only reducing operational costs but also providing a promising path for improving health outcomes for billions around ...
Electrospinning technique converts polymeric solutions into nanoscale fibers using an electric field and can be used for various biomedical and clinical applications. Extracellular vesicles (EVs) are cell-derived small lipid vesicles enriched with biological cargo (proteins and nucleic acids) potential therapeutic applications. In this review, we discuss extending the scope of electrospinning ...