Thank you for visiting nature.com. You are using a browser version with limited support for CSS. To obtain the best experience, we recommend you use a more up to date browser (or turn off compatibility mode in Internet Explorer). In the meantime, to ensure continued support, we are displaying the site without styles and JavaScript.
- View all journals
- My Account Login
- Explore content
- About the journal
- Publish with us
- Sign up for alerts
- Open access
- Published: 26 November 2020
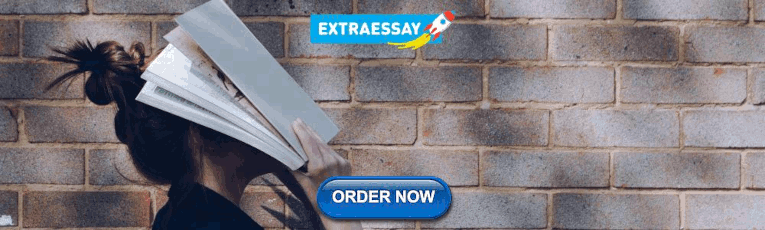
Performance evaluation of a large-scale thermal power plant based on the best industrial practices
- Yousef S. H. Najjar 1 &
- Amer Abu-Shamleh ORCID: orcid.org/0000-0002-2553-731X 1
Scientific Reports volume 10 , Article number: 20661 ( 2020 ) Cite this article
17k Accesses
6 Citations
Metrics details
- Diesel fuel
- Natural gas
The aim of this study is to assess and evaluate the performance of a large-scale thermal power plant (TPP). The performance rating was conducted in compliance with the statistical principles. The data for this analysis were obtained for a TPP with an installed capacity of 375 MW during a span of 8 years (2010–2017). Four parameters were used to evaluate the performance of the TPP including the availability, the reliability, the capacity factor, and the thermal efficiency. These parameters were calculated using a set of equations and then compared to the international best practices and target values. The results indicate that approximately 91% of the expected capacity was available throughout the studied period against the industry best practice of 95%. However, the average TPP’s reliability was found to be approximately 95% against the target value of 99.9%. Furthermore, the average capacity factor throughout the studied period is 70% as against the international value of 40–80%. Moreover, the thermal efficiency of the TPP is 40% against the target value of 49%. Due to the outage hours and malfunctions, the power losses throughout the studied period reached 846 MW. Overall, the analysis indicates that the studied TPP is not within the scope of the best industrial practices.
Similar content being viewed by others
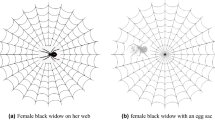
Greenhouse gases emission reduction for electric power generation sector by efficient dispatching of thermal plants integrated with renewable systems
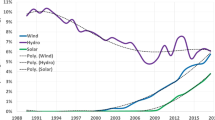
Techno-economic performances of future concentrating solar power plants in Australia
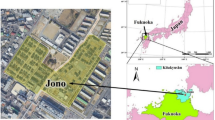
Analysis of the potential application of a residential composite energy storage system based on a double-layer optimization model
Introduction.
The gas turbine (GT), also known as the combustion turbine, is a rotary motor that removes energy from a hot gas flow generated in a stream of compressed air by combustion of gas or fuel oil. The GT has a radial or axial flow air compressor mechanically connected with an upstream turbine and combustion chamber. Energy is released by the mixing and ignition of compressed air into the combustion chamber (combustor) 1 . Energy is generated in shaft power by GTs and used to power generators and other machinery. GTs have been recognized as prime movers for reliable baseload applications and they are being increasingly used world wide 2 , 3 .
GTs have earned a privileged position among other electrical generation technologies due to its high efficiency and reliability, particularly when incorporated with combined cycle 4 . GTs are also known for their flexibility and regular availability 4 . However, the performance of the GT is influenced by both the efficiency of components and the turbine inlet temperature 5 , 6 . In addition, the operation of GTs and combined-cycle operations is predominantly affected by the long-term operation. In addition, the output of the gas-turbine engine has relatively poor performance at part-load, and power output ( \({P}_{\mathrm{out}}\) ) deteriorates during hot seasons 7 , 8 . Najjar et al. 9 studied the performance diagnostics and the degradation of the GT cycle using actual data obtained from a combined-cycle power plant throughout 2 years of operation. It has been concluded that the degradation of the GT increases with temperature and load over time. In this context, the performance ratings of thermal power plants (TPPs) including reliability, availability, capacity factor, and efficiency, are expected to flocculate with the operation time.
CCGT is one type of power plant that directs the exhaust gas of the GT over a heat exchanger which generates steam at various levels of pressure 10 . The performance analysis of the combined-cycle gas turbine (CCGT) focuses mainly on evaluating the power efficiency of the plant 11 . The efficiency of a plant has a definite economic significance because heat inputs are the energy that is to be purchased at high temperatures, and net energy production is the return on the energy that is purchased. The GT running at lower inlet temperatures of the turbine produces low performance thus, lower efficiency 12 . The lower efficiency of the GT means that low \({P}_{\mathrm{out}}\) is produced. Several factors affecting the efficiency of TPPs in general, these include age, fuel type, capacity factor, and heat sink system 13 .
Sabouhi et al. 14 , investigated the reliability of a CCGT power plant using a developed model. The analysis involved modeling both GT and steam turbine power plants from an engineering system perspective, which provided the necessary data to estimate the reliability of the CCGT. Overall, the results point out to the most important components that help in selecting convenient strategies for the CCGT power plants 15 . On the other hand, Kolawole et al. 16 studied the availability, reliability, and capacity factor of a power generating plant using its historical data. It has been concluded that plant unavailability, the grid constraints, and gas restriction prevented the power plant from running at the maximum continuous rating. However, they suggested that in order to enhance the power supply, there is a demand for better maintenance, adequate gas supply, and the examination of the distribution and transmission units.
Lamfon et al. 17 studied the performance of a GT with a capacity of 23.7 MW operated at an ambient temperature between 30 and 45 °C. They reported an 11% improvement in the net \({P}_{\mathrm{out}}\) when the inlet of the GT engine is provided with cold air. However, an increase of 11% in the net \({P}_{\mathrm{out}}\) is also reported at an ambient temperature of 30 °C. This was based on the International Standard Organization (ISO) rated condition along with a 2% increase in \(\eta\) with a 2% decrease in specific fuel consumption. However, Ameri et al. 18 investigated the variation of ambient temperature on the \({P}_{\mathrm{out}}\) . It has been pointed out that if the ambient temperature drops from 34.2 °C to the ISO-rated condition an average increase in the \({P}_{\mathrm{out}}\) by as much as 11.3% can be achieved. It has also been reported that the \({P}_{\mathrm{out}}\) efficiency decreased by 0.74% when the ambient air temperature increased by 1 °C. In another similar study, Mohanty et al. 19 concluded that the net \({P}_{\mathrm{out}}\) decrease by 10% when the inlet air temperature increases by 30% from the ISO-rated conditions. Nevertheless, for small-scale GTs, this incline in the net \({P}_{\mathrm{out}}\) can be much greater. In addition, the study showed that raising the ambient temperature by 1 °C would result in about a 1% drop in the rated capacity of the GT.
Given the important role of energy in economic development in a country and the anticipated substantial future requirements, it is a key choice to conserve energy and make productive usage. The aim of this paper is to report on an evaluation method to assess the performance of a TPP in terms of availability, reliability, capacity factor, and thermal efficiency, for a period of 8 years (from 2010 to 2017). The proposed evaluation methodology will help in the performance diagnostics of similar power plants. Moreover, this evaluation is useful for linking maintenance to the overall performance of the power plant, thus, helping in maintenance scheduling.
Power plant description
The plant is situated in the northern part of Jordan, 70 km from the capital Amman. The region where the plant is located has a warm climate with average ambient temperature and relative humidity of 18 °C and 48% respectively. The plant is located within a rural region approximately 832 m above sea level.
The power plant consists of two simple-cycle GTs with a rated capacity of 30 MW each and a 297 MW CCGT comprised of two GTs which are nominally rated at 100 MW each and one ST with a rated capacity of 97 MW. The plant utilizes two types of fuels namely, diesel oil and natural gas and uses dry cooling fans for cooling. Figure 1 shows a schematic diagram of a CCGT.
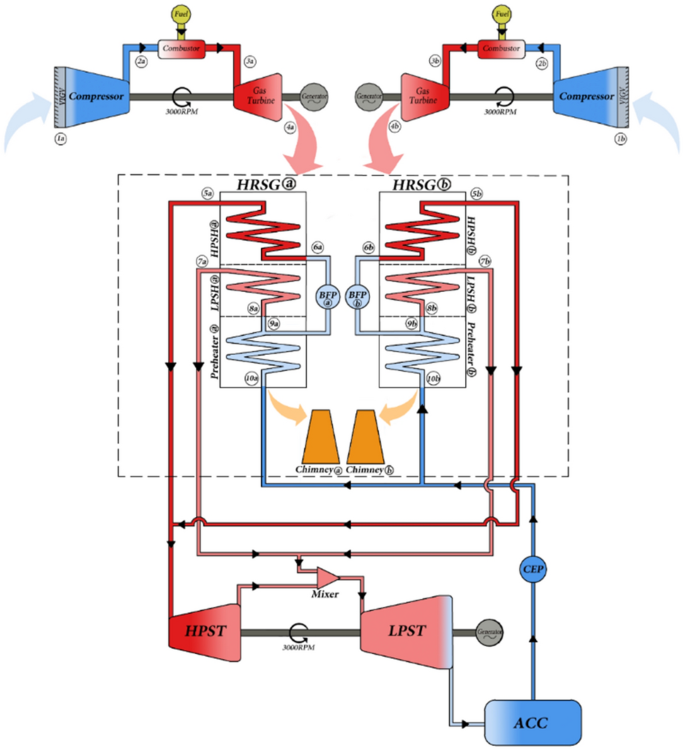
A schematic of process flow throughout a CCGT. Reprinted from Sustainable Energy Technologies and Assessments, 37, Najjar, Y. S. H., Alalul, O. F. A. & Abu-Shamleh, Degradation analysis of a combined cycle heat recovery steam generator under full and part load conditions. Sustainable Energy Technologies and Assessments, 100,587, 2020, with permission from Elsevier.
Data collection
The data of the power plant were obtained for a period of 8 years (2010–2017) from CEGCO annual reports 20 , 21 . Throughout the studied period, several major malfunctions occurred to the power plant. Though minor failures were also observed during the extended period, the major failures were observed for the years 2010, 2012, and 2013. These malfunctions affected the overall performance of the power plant and caused some of the generation units to be out of service for several days. The total number of days the malfunctioned units were out of service throughout the studied period is 206. Table 1 summarizes the major failures the power plant was exposed to during the period of the study.
The specific data that was used to evaluate the power plant performance includes, total installed capacity (MW), power output (MW), generated electrical energy ( \({E}_{g}\) ) (MWh), running hours (h), heat supply (MW), and heat rate (HR) (kJ/kWh). Table 2 shows the data of the power plant throughout the studied period.
Performance analysis
The performance evaluation was carried out based on statistical principles. The evaluation was carried out based on four key parameters namely, availability, reliability, capacity factor, and thermal efficiency. These parameters are calculated based on the data of the power plant and then compared to the best industrial practices and target values.
The forced outage factor (FOF) and the planned outage factor (POF) were both used to calculate the annual running hours of the TPP by subtracting the number of hours per year from both. FOF is defined as the shutdown of the plant as a result of undesired occurrences, whereas POF is defined as the prescheduled shutdown as for routine maintenance 22 . Equations 1 – 9 were used to calculate the identified 4 parameters. The calculated FOF and POF are presented in Table 3 .
The running hours of the power plant per each year can be calculated using the following equation:
where \(FOH\) is the forced outage hours. and \(POH\) is the planned outage hours.
The forced outage factor can be calculated as follows:
The planned outage factor can be calculated as follows:
The power output of the plant in MW was obtained as follows:
The availability of the power plant is calculated using the following equation:
The reliability of the power plant is calculated using the following equation:
The capacity factor of the power plant is calculated using the following equation:
The thermal efficiency ( \({\eta }_{th}\) ) of the power plant throughout the studied period is calculated as follows:
Finally, the HR of the power plant can be calculated as follows:
Results and discussion
The calculated values for each rating parameter across the studied period are shown in Table 4 . These values are fluctuated based on the performance of the TPP during the years. Ideally, the total generated power of the TPP during the 8 years can be calculated by multiplying the total installed capacity by the number of the years. In this case, the total generated power in MW should be 2856 MW, however, the actual generated power is less than that, due to the TPP being operated at part load conditions, along with other technical reasons including outage hours and malfunctions. The actual power generated throughout the studied period is approximately 1991.6 MW, meaning that 864.4 MW was lost. Overall, this operating loss does not mean that the plant is not achieving its purpose as most of the power plants are expected to have such huge losses during the years. In this context, the performance analysis is used to decide whether these losses are significant or not. Figure 2 illustrates the actual power generated to the installed generated power.
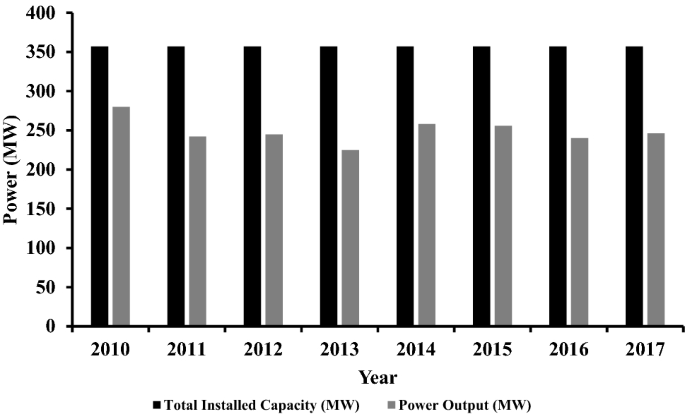
The generated power by the TPP throughout (2010–2017).
The availability factor of a power plant is the amount of time that it is able to produce electricity over a certain period, divided by the amount of time in the period 23 . The availability factor depends on the operation of the power plant, the fuel type, and the design of the plant. In this study, the availability of the studied TPP varies from 82.3 to 97.7%. Considering that the best industrial practices designate that GTs have a relatively high availability factor of 95% 24 , the TPP, on average, is not within the target value. Figure 3 shows the variation of the availability factor over the studied period.
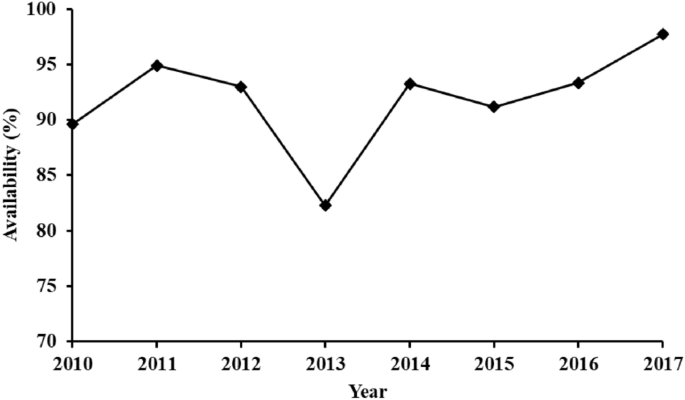
The availability factor of the TPP throughout (2010–2017).
The reliability analysis is an important step in the evaluation of CCGTs and plays a significant role in the operation of the plant in terms of maintenance scheduling 25 . However, the reliability factor of a power plant is mainly dependent upon the FOH and can be calculated by dividing the FOH by the actual time of operation. Over the studied period, the reliability of the TPP ranged between approximately 82.4% and 99.8% as shown in Fig. 4 . The lowest value has been obtained for the year 2013 due to one GT being out of service for a relatively large amount of time as mentioned in Table 1 . Nevertheless, the TPP’s average reliability was found to be 95.4%. Since there is no mentioning of the best industrial range of reliability for CCGTs in the literature, this percentage was compared to the starting reliability value of 99.9% 26 . This means that the reliability of the power plant is not within the best industrial practices. The high average percentage obtained can be attributed to the annual percentage of hours due to forced outage and the planned outage is around 4%.
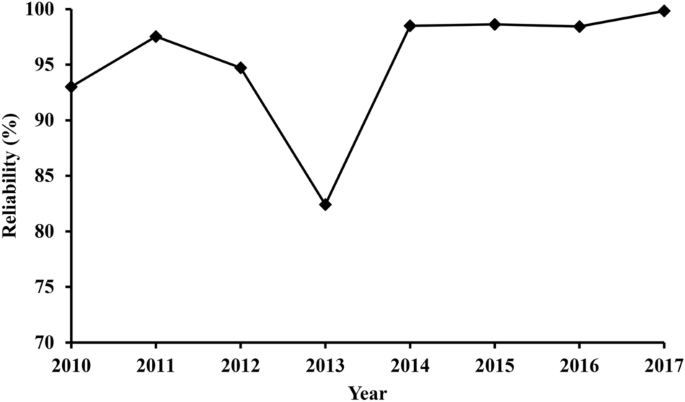
The reliability factor of the TPP throughout (2010–2017).
The capacity factor of a power plant is essentially a measure of its overall utilization 27 . For a power plant, the capacity factor can be calculated by dividing the actual electricity produced by its maximum possible electricity output throughout a certain period of time 28 . The average capacity factor of the TPP over the studied period is 70% with a minimum value of 65.6% obtained for 2013 and a maximum value of 76% for the year 2010. The flocculation of the capacity factor can be attributed to the age of the plant, outages, operation and maintenance, and weather conditions. Based on the international values, the average capacity factor of a power plant within a specific period should not be less than 65% 26 , meaning the power plant is within the normal ranges. Figure 5 shows the graph of the capacity factor throughout the studied years.
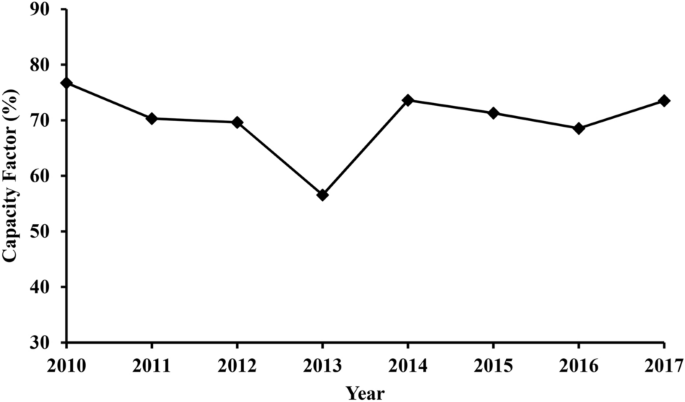
The capacity factor of the TPP throughout (2010–2017).
The thermal efficiency of the power plant is defined as the power output of the plant divided by the heat supplied. The thermal efficiency mainly depends on the heat value of the fuel used and the temperature. As the inlet temperature of the turbine increases, the thermal efficiency increases 29 . The thermal efficiency of the studied TPP throughout (2010–2017) is shown in Fig. 6 . The average thermal efficiency of the TPP is approximately 39.9%. The maximum obtained value is approximately 40.8% for both 2012 and 2016, while the minimum value is approximately 39.8% for the year 2013. Although the large malfunctions that occurred in 2013 do not directly affect the thermal efficiency, the cut in the fuel supply as a result does. Since the power plant is composed of two units (simple and combined), the obtained efficiency was compared to the target value of 49%. This value is the average between the approximate thermal efficiency of simple cycle gas power plants which is in the range of 35–42% 30 , and that average value for CCGTs which is 60% 31 .
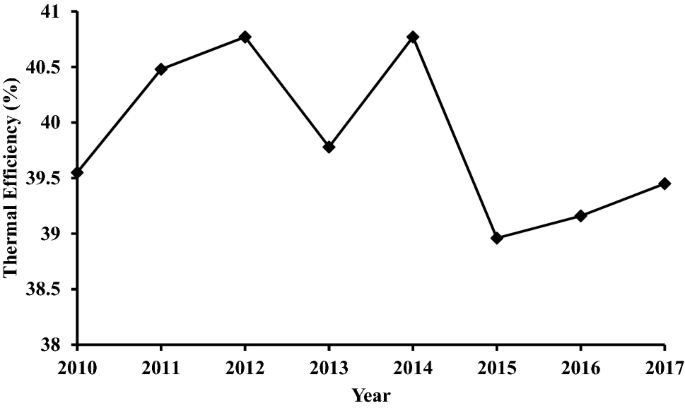
The thermal efficiency of the TPP throughout (2010–2017).
GTs are designed for standard air conditions. Nevertheless, the operating times are much greater at off-design conditions than at site conditions. When a GT operates at site environmental conditions different from the ISO, a distinction can be found between the actual power produced by a GT and the design-rated power marked on the GT. A comprehensive study and recording of operating data have shown that the ambient temperature is related to direct degradation of the GT capacity 32 . The GT loses 1% of its \({\eta }_{th}\) and 1.47 MW of gross capacity for each 1 °C increase in the ambient temperature above the ISO limit.
The GT's power generation output is dependent on the turbine inlet temperature. The temperature of the turbine inlet plays an important role in the performance of a cycle system. The efficiency of the component and the working temperature of the turbine affect the GT performance. The overall efficiency of the CCGT is mainly dependent on the compressor pressure ratio and turbine inlet temperature 33 . The key performance parameters are summarized and shown in Table 5 . These parameters are compared to the industry's best practices and target values.
Conclusions
In this study, a large-scale TPP was evaluated based on statistical principles. The evaluation was based on collected data from an actual power plant for a period of 8 years. The main performance parameters that were studied include availability, reliability, capacity factor, and thermal efficiency. These parameters were compared to the best industrial practices and target values. The average availability and reliability of the TPP throughout the studied period were found to be approximately 91% and 95% respectively. In addition, the average capacity factor and thermal efficiency over the studied period were found to be 70% and 40% respectively. The power losses out of the total generated electrical power over the studied period reached 864.4 MW. Several major malfunctions were reported over the studied period which caused the TPP to be out of service for a number of days. These malfunctions are linked to the overall performance of the TPP and the evaluated parameters. Overall, the analysis indicates that the plant is not within the context of the best industrial practices. Moreover, the evaluation methodology followed in this research can be useful in building maintenance schedules for such plants.
Recommendations
The CCGT efficiency and reliability can be improved considerably. The following are suggested ways to improve the performance of such a plant:
Increasing the inlet temperature of the turbine, taking into consideration the building material of the turbine can withstand high temperatures or by replacing the current parts with others that can withstand high temperatures. For 56 °C, the work output rises by around 10%, and the overall efficiency also increases by 1.5% 34 .
Suitable maintenance and cleaning of the inlet filters of the compressors. Dirty and poorly maintained filters cause significant efficiency loss as the compressor blades suffer clogging and damage. In addition, the pressure drop can be caused by dirty filters. Thus, Regular part checks and cleaning will boost plant performance and reliability.
Abbreviations
Combined-cycle gas turbine
Generated electrical energy (MWh)
Forced outage factor (%)
Forced outage hour (h)
Gas turbine
Heat rate (kJ/kWh)
International Organization for Standardization
Planned outage factor (%)
Planned outage hour (h)
Power output (MW)
Thermal power plant
Thermal efficiency (%)
Air compressor inlet
Combustor outlet
Gas turbine inlet
Gas turbine outlet
Inlet of high-pressure heat recovery steam generator
Outlet of high-pressure heat recovery steam generator
Inlet of low-pressure heat recovery steam generator
Outlet of low-pressure heat recovery steam generator
Inlet of heat recovery steam generator preheater
Outlet of heat recovery steam generator preheater
Ukwamba, S. I., Orhorhoro, E. K. & Omonoji, A. A. Performance evaluation of a simple gas turbine power plant using vapour absorption chiller. IOSR J. Mech. Civ. Eng. 15 , 13–18 (2018).
Google Scholar
Najjar, Y. S. H., Akyurt, M., Al-Rabghi, O. M. & Alp, T. Cogeneration with gas turbine engines. Heat Recov. Syst. CHP 13 , 471–480 (1993).
Article CAS Google Scholar
Najjar, Y. S. H. & Zaamout, M. S. Comparative performance of closed cycle gas turbine engine with heat recovery using different gases. Heat Recov. Syst. CHP 12 , 489–495 (1992).
Mohamed, O. & Khalil, A. Progress in modeling and control of gas turbine power generation systems: a survey. Energies 13 , 2358 (2020).
Article Google Scholar
Basrawi, F., Yamada, T., Nakanishi, K. & Naing, S. Effect of ambient temperature on the performance of micro gas turbine with cogeneration system in cold region. Appl. Therm. Eng. 31 , 1058–1067 (2011).
Najjar, Y. S. H., Abubaker, A. M. & El-Khalil, A. F. S. Novel inlet air cooling with gas turbine engines using cascaded waste-heat recovery for green sustainable energy. Energy 93 , 770–785 (2015).
Najjar, Y. S. H. & Abubaker, A. M. Indirect evaporative combined inlet air cooling with gas turbines for green power technology. Int. J. Refrig 59 , 235–250 (2015).
Najjar, Y. S. & Zaamout, M. S. Enhancing gas-turbine engine performance by means of the evaporative regenerative cycle. J. Inst. Energy 69 , 2–8 (1996).
CAS Google Scholar
Najjar, Y. S. H., Alalul, O. F. A. & Abu-Shamleh, A. Degradation analysis of a heavy-duty gas turbine engine under full and part load conditions. Int. J. Energy Res. 44 , 4529–4542 (2020).
Najjar, Y. S. H., Alalul, O. F. A. & Abu-Shamleh, A. Steam turbine bottoming cycle deterioration under different load conditions. Therm. Sci. Eng. Prog. 20 , 100733 (2020).
Orhorhoro, E. K., Polytechnic, D. S., Orhorhoro, O. W. & Polytechnic, D. S. Simulation of air inlet cooling system of a gas turbine power. ELK Asia Pac. J. Appl. Therm. Eng. 1 , 2394-0433 (2016).
Akyurt, M., Lamfon, N. J., Najjar, Y. S. H., Habeebullah, M. H. & Alp, T. Y. Modeling of waste heat recovery by looped water-in-steel heat pipes. Int. J. Heat Fluid Flow 16 , 263–271 (1995).
Aziz, N. L. A. A., Yap, K. S. & Bunyamin, M. A. A hybrid fuzzy logic and extreme learning machine for improving efficiency of circulating water systems in power generation plant. in IOP Conference Series: Earth and Environmental Science , Vol. 16 (2013).
Sabouhi, H., Abbaspour, A., Fotuhi-Firuzabad, M. & Dehghanian, P. Reliability modeling and availability analysis of combined cycle power plants. Int. J. Electr. Power Energy Syst. 79 , 108–119 (2016).
Ibrahim, T. K. et al. The optimum performance of the combined cycle power plant: a comprehensive review. Renew. Sustain. Energy Rev. 79 , 459–474 (2017).
Kolawole, A., Agboola, O. O., Ikubanni, P. P., Raji, O. G. & Okechukwu Osueke, C. Reliability and power loss analysis: a case study of a power plant in Nigeria. Cogent Eng. 6 , 1–13 (2019).
Lamfon, N. J., Najjar, Y. S. H. & Akyurt, M. Modeling and simulation of combined gas turbine engine and heat pipe system for waste heat recovery and utilization. Energy Convers. Manag. 39 , 81–86 (1998).
Ameri, M. & Hejazi, S. H. The study of capacity enhancement of the Chabahar gas turbine installation using an absorption chiller. Appl. Therm. Eng. 24 , 59–68 (2004).
Mohanty, B. & Paloso, G. Enhancing gas turbine performance by intake air cooling using an absorption chiller. Heat Recov. Syst. CHP 15 , 41–50 (1995).
Central Electricity Generating Company (CEGCO). Annual Report 2017 . (2017).
Central Electricity Generating Company (CEGCO). Annual Report 2013 . (2013).
Graf, R. F. O. in Modern Dictionary of Electronics , 7th edn. (ed. Graf, R. F.) 514–533 (Newnes, 1999). https://doi.org/10.1016/B978-0-08-051198-6.50020-5
Institute of Electrical and Electronics Engineers. Std 762-2006—IEEE Standard Definitions for Use in Reporting Electric Generating Unit Reliability, Availability, and Productivity (2006).
Oyedepo, S. O., Fagbenle, R. O., Adefila, S. S. & Adavbiele, S. A. Performance evaluation and economic analysis of a gas turbine power plant in Nigeria. Energy Convers. Manag. 79 , 431–440 (2014).
Dev, N., Kachhwaha, S. S. & Attri, R. Development of reliability index for combined cycle power plant using graph theoretic approach. Ain Shams Eng. J. 5 , 193–203 (2014).
Oyedepo, S. O., Fagbenle, R. O. & Adefila, S. S. Assessment of performance indices of selected gas turbine power plants in Nigeria. Energy Sci. Eng. 3 , 239–256 (2015).
Morales Pedraza, J. Chapter 4—Current Status and Perspective in the Use of Coal for Electricity Generation in the North America Region. In Conventional Energy in North America (ed. Morales Pedraza, J.) 211–257 (Elsevier, Amsderdam, 2019). https://doi.org/10.1016/B978-0-12-814889-1.00004-8 .
Chapter Google Scholar
Neill, S. P. & Hashemi, M. R. Chapter 1—Introduction. In Fundamentals of Ocean Renewable Energy (eds Neill, S. P. & Hashemi, M. R.) 1–30 (Academic Press, London, 2018). https://doi.org/10.1016/B978-0-12-810448-4.00001-X .
Alfellag, M. A. A. Parametric investigation of a modified gas turbine power plant. Therm. Sci. Eng. Prog. 3 , 141–149 (2017).
Willnow, K. Energy Efficiency Technologies ANNEX III Technical Report for Thermal Power Plants . WEC Knowledge Network (2013).
da Matto, H. A. et al. Combined cycle performance evaluation and dynamic response simulation. J. Aerosp. Technol. Manag. 8 , 491–497 (2016).
Najjar, Y. S. H., Alalul, O. F. A. & Abu-Shamleh, A. Degradation analysis of a combined cycle heat recovery steam generator under full and part load conditions. Sustain. Energy Technol. Assess. 37 , 100587 (2020).
Najjar, Y. S. A cryogenic gas turbine engine using hydrogen for waste heat recovery and regasification of LNG. Int. J. Hydrog. Energy 16 , 129–134 (1991).
William, O. E. Performance analysis of gas turbine power (a case study of Delta III GT9 Transcorp Gas Turbine Power Plant, Ughelli, Nigeria). Global Scientfic Journals 44–55 (2018).
Download references
Acknowledgements
The authors received no specific funding for this work.
Author information
Authors and affiliations.
Mechanical Engineering Department, Jordan University of Science and Technology, Irbid, Jordan
Yousef S. H. Najjar & Amer Abu-Shamleh
You can also search for this author in PubMed Google Scholar
Contributions
Yousef S. H. Najjar: Supervision. Amer Abu-Shamleh: Writing—original draft, methodology, investigation, validation, Writing—review & editing.
Corresponding author
Correspondence to Amer Abu-Shamleh .
Ethics declarations
Competing interests.
The authors declare no competing interests.
Additional information
Publisher's note.
Springer Nature remains neutral with regard to jurisdictional claims in published maps and institutional affiliations.
Rights and permissions
Open Access This article is licensed under a Creative Commons Attribution 4.0 International License, which permits use, sharing, adaptation, distribution and reproduction in any medium or format, as long as you give appropriate credit to the original author(s) and the source, provide a link to the Creative Commons licence, and indicate if changes were made. The images or other third party material in this article are included in the article's Creative Commons licence, unless indicated otherwise in a credit line to the material. If material is not included in the article's Creative Commons licence and your intended use is not permitted by statutory regulation or exceeds the permitted use, you will need to obtain permission directly from the copyright holder. To view a copy of this licence, visit http://creativecommons.org/licenses/by/4.0/ .
Reprints and permissions
About this article
Cite this article.
Najjar, Y.S.H., Abu-Shamleh, A. Performance evaluation of a large-scale thermal power plant based on the best industrial practices. Sci Rep 10 , 20661 (2020). https://doi.org/10.1038/s41598-020-77802-8
Download citation
Received : 10 April 2020
Accepted : 10 November 2020
Published : 26 November 2020
DOI : https://doi.org/10.1038/s41598-020-77802-8
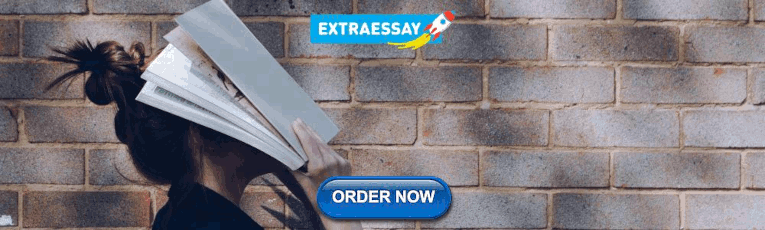
Share this article
Anyone you share the following link with will be able to read this content:
Sorry, a shareable link is not currently available for this article.
Provided by the Springer Nature SharedIt content-sharing initiative
By submitting a comment you agree to abide by our Terms and Community Guidelines . If you find something abusive or that does not comply with our terms or guidelines please flag it as inappropriate.
Quick links
- Explore articles by subject
- Guide to authors
- Editorial policies
Sign up for the Nature Briefing newsletter — what matters in science, free to your inbox daily.

Academia.edu no longer supports Internet Explorer.
To browse Academia.edu and the wider internet faster and more securely, please take a few seconds to upgrade your browser .
Enter the email address you signed up with and we'll email you a reset link.
- We're Hiring!
- Help Center
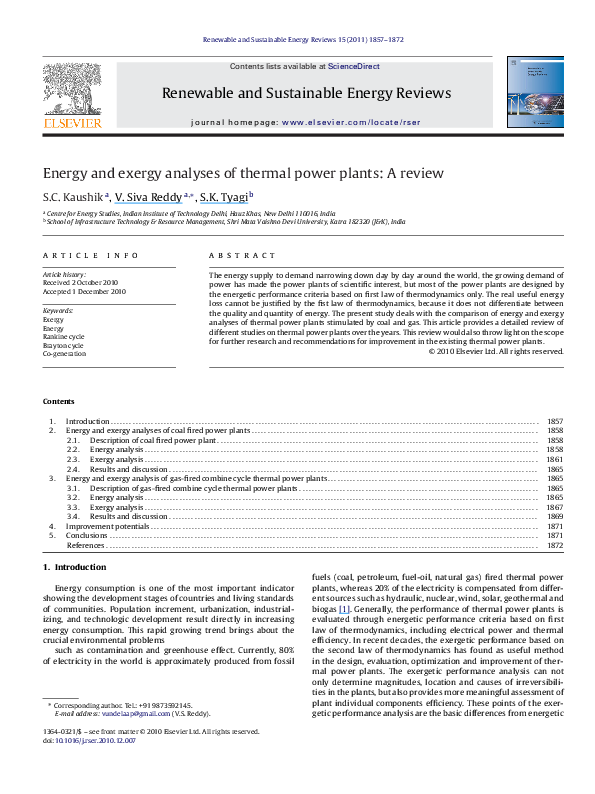
Energy and exergy analyses of thermal power plants: A review

2011, Renewable and Sustainable Energy Reviews
Related Papers
sadanand nautiyal
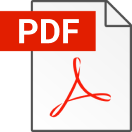
Farhana Alam
This paper addresses the energetic and exergetic efficiencies and losses in different components and the overall system of a 250 MW coal based thermal power plant. The plant is operated under Bangladesh Power Development Board (BPDB), which is located at Barapukuria, Bangladesh and consists of 2 units (2 x 125 MW). The analyses have been carried out by considering design data for different designed and loading conditions of the plant. The overall energy efficiencies of the plant are 35.48%, 56.77%, 70.96% and 75.67% and the overall exergy efficiencies were 44.25%, 33.31%, 30.78 % and 30.21% for 50%, 80%, 100% and 106% loading conditions respectively for the design data. However, the overall energy and exergy efficiencies of the power plant during operation are 39.2%, 46.6% and 27.9 %, 27.2% for 57% and 67% loading conditions respectively, which are lower than the design value. The distribution of the exergy losses in power plant components has been assessed to locate the process irr...
6th BSME International Conference on Thermal Engineering (ICTE 2014)
AKM Nazrul Islam , Farhana Alam
This paper addresses the energetic and exergetic efficiencies and losses in different components and the overall system of a 250 MW coal based thermal power plant. The plant is operated under Bangladesh Power Development Board (BPDB), which is located at Barapukuria, Bangladesh and consists of 2 units (2 x 125 MW). The analyses have been carried out by considering design data for different designed and loading conditions of the plant. The overall energy efficiencies of the plant are 35.48%, 56.77%, 70.96% and 75.67% and the overall exergy efficiencies were 44.25%, 33.31%, 30.78 % and 30.21% for 50%, 80%, 100% and 106% loading conditions respectively for the design data. However, the overall energy and exergy efficiencies of the power plant during operation are 39.2%, 46.6% and 27.9 %, 27.2% for 57% and 67% loading conditions respectively, which are lower than the design value. The distribution of the exergy losses in power plant components has been assessed to locate the process irreversibility. The comparison between the energy losses and the exergy losses of the individual components of the plant shows that the maximum energy losses (~49.92%) occur in the condenser, whereas the maximum exergy losses (~68.27%) occur in the boiler. Exergy analysis can be particularly effective in identifying ways to optimize the performance of existing operations and designing the plant while energy balance gives heat transfer between the system and its surrounding.
Journal of Scientific and Engineering Research , 201 7 , 4 ( 7 ): 2 15 - 2 29
AKM Nazrul Islam
This article aims to identify the energetic and exergetic efficiencies and the losses in different components of a 250 MW coal based thermal power plant. The analysis has been carried out by considering the design data as well as the operational data at varying load conditions. The distribution of the exergy losses in power plant components has been assessed to locate the process irreversibility. Basically, the power plants are designed based on first law of thermodynamics that does not afford to calculate useful energy losses of the system due to the quality and quantity of energy difference. This study deals with the comparison of energy and exergy analyses of the Barapukuria coal fired thermal power plant of Bangladesh, which deals with both first and second law of thermodynamics. Operation and maintenance decisions based on exergy analysis (second law analysis) would be more effective for operation of the thermal power plant.
International Journal of Engineering Research and Technology (IJERT)
IJERT Journal
https://www.ijert.org/exergy-analysis-of-thermal-power-plant-a-review https://www.ijert.org/research/exergy-analysis-of-thermal-power-plant-a-review-IJERTV2IS70048.pdf Power generation industry plays a key role in the economic growth of the country. The energy supply to demand narrowing down day by day around the world, the growing demand of power has made the power plants of scientific interest, but most of the power plants are designed by the energy performance criteria based on first law of thermodynamics only. The real useful energy loss cannot be justified by the fist law of thermodynamics, because it does not differentiate between the quality and quantity of energy. The present study deals with the exergy analyses of thermal power plants stimulated by coal and provide a detailed review of different studies on thermal power plants over the years. This review will identify major sources of losses and exergy destruction in the power plant. It will provide ways and means to improve the system performance and reduce environment impact.
Marko Mancic
The global trend of growing energy demand, accompanied by the dependency on fossil fuels and their impacts, provokes continuous interests for analyzing equipment efficiency and revision of existing energy production sites. This paper focuses on thermodynamic analysys of a coal-fired power plant "Kolubara - block A5", with respect to the concept of energy losses, production of entropy and exergy destruction.Most significant energy losses occur in the condenser where they are lost to the environment, whereas greatest exergy destruction is found in the boiler. The results of energy and exergy performance of the plant are calculated for maximum load and 75% of the maximum load. Each of the system components is modeled as a "black box", and the energy and exergy balances are determined according to operation data. Thermal efficiency, based on the specific heat input to the steam, was calculated and found to be 32.1% and 33.1%, while the exergy efficiency of the power ...
eSAT Journals
In this present investigation exergy and energy efficiencies of a coal fired 250 MW thermal power plant operating in eastern part of India are determined both for 100% and 90% load based on actual operating data. The efficiencies are evaluated for the overall plant as well as for different equipments like boiler, turbine, all feed water heaters and condenser. Similarly effectiveness of the feed water heaters is evaluated for both the loads. Exergy destruction % for each of the equipment are also given for clear understandingof the loss of available energy due to irreversibilities involved in the processes for each equipment and the whole plant. It is observed that a major irreversibility or, exergy destruction takes place at boiler though 1st law energy efficiency is quite high. This signifies that there might have further scope of improvement in this equipment. Similar analyses are carried out for other equipments. The results obtained in present analysis are compared with those of other investigators.
Dr. ASHWINI KUMAR
IJSRD - International Journal for Scientific Research and Development
In this paper energy and exergy analysis method for thermal power plant and analysis carried out on 55 MW coal base thermal power plant of DCM – Shriram Ltd. In the present, increasing demand of power has made the power plants of scientific interest, but majority of the power plants are designed by the energetic performance criteria based on first law of thermodynamics only. The first law of thermodynamics does not give all the ideas about the useful energy loss because the difference between the quality and quantity cannot be justified by it. In the energy analysis we can presents only quantitative results while in exergy analysis one can presents qualitative results about actual energy consumption. In this analysis it is shown that exergy efficiency is less at each and every point of power plant’s component compare to energy efficiency. It also presents major losses of available energy at combustor, super heater, economizer and air-pre heater section. In this article it is also shown energy efficiency, exergy efficiency, exergy destruction and energy losses comparison charts.
IJESRT Journal
Steadily growing demand of power and heavily dependence on conventional sources like coal and gas for power generation has made it imperative to utilize these resources efficiently and economically. The conventional steam power plant designs are normally based on the energetic performance of plant components viz. boiler, turbine etc. First law based energetic performance analysis is not sufficient to know about the complete utilization of energy as it does not provide any information regarding quality degradation during transformation process. In this research paper concept of exergetic performance analysis i.e. application of first and second law of thermodynamics together, has been utilized to identity the location/components where major energy and exergy losses takes place and also to determine the extent of losses. In the present study complete first and second law analysis of a 250MW coal fired thermal power plant at Korba, Chhattisgarh, India, is carried out at three different loads. The energy and exergy efficiency of the components have been determined. The energy efficiency of the plant is found to be 38.5%, 37.54% and 34.63% respectively for the plant operation at 250MW, 200MW and 125MW. A similar trend is seen in exergetic performance and exergy efficiency is recorded as 36.32%, 34.41% and 32.67% respectively at three loads mentioned above. It is observed that at the full load of 250MW the maximum energy loss (46.26% of the input) among all the components is taking place in the condenser which is the 72.46% of total energy loss whereas the maximum exergy loss of around 50% of the input takes place in the boiler and this contributes to 76.67% of total exergy loss. Both on the basis of quantity and percent exergy loss, boiler is the key component showing huge potential for saving. This study suggests that the possible measures should be taken to avoid the quality degradation of energy in boiler i.e. in the zone of higher temperature gradient and operation of the plant closed to the full load should be ensured for better performance.
RELATED PAPERS
Journal of Business and Management Studies
Danu Setiawan
The Journal of Thoracic and Cardiovascular Surgery
Randall Wolf
Ecotoxicology and Environmental Safety
Abhik Gupta
Death Studies
Cecilia Chan
Case Reports in Critical Care
Rafael Jorge
karen portilla
Frontiers in Education
Barbara Wasson
Bulletin of the American Physical Society
Murat Aktas
Jasmin Reyes
如何购买sfu毕业证 西蒙菲莎大学毕业证研究生文凭证书托业成绩单原版一模一样
Danilyn Rutherford
Journal of Engineering Research
Mennatullah Hendawy
The Journal of Urology
Laureano Rangel
Water Research
Toxicological Sciences
Michael Hughes
Communitarian Letter #12
Margaret Stout
Journal of endodontics
Shebli Mehrazarin
SSRN Electronic Journal
Enrique ter Horst
Groundwater
Domenico Pianese
Chemical Communications
Bharathi Srinivasan
RELATED TOPICS
- We're Hiring!
- Help Center
- Find new research papers in:
- Health Sciences
- Earth Sciences
- Cognitive Science
- Mathematics
- Computer Science
- Academia ©2024
Thermal Power Generation
- First Online: 31 May 2016
Cite this chapter
- Takao Nakagaki 5
1864 Accesses
Thermal power plants will be a promising power source even in the 2050s, because they can generate a vast amount of electricity with low cost, high reliability, and stability. The plants will also have strong flexibility and controllability to compensate the gap between power demand and supply with coexistence of a certain amount of unstable renewable power sources. Coal, oil, and liquefied natural gas (LNG) are mainly used for thermal power generation in the electricity business and are evenly mixed because of energy security, the so-called best mix. After the Great East Japan Earthquake, thermal power generation of all electric companies drastically increased by 164 TWh (+33.9 %), to compensate their nuclear power generation.
Japan now has world-class, excellent thermal power technologies with heavy-duty steam turbines under the ultra-supercritical (USC) steam condition and high-efficiency 1600 °C class LNG-fired gas turbines. By 2030, if technical development projects of “advanced USC” and 1700 °C class gas turbines are completed successfully, 46 and 57 % net thermal efficiencies at the sending end (higher heating value, HHV) will be achieved in commercial power plants, respectively. By 2050, the integrated coal gasification fuel cell combined cycle (IGFC) is expected to appear, and CO 2 capture and storage (CCS) will move forward with full-scale implementation. However, introduction costs of these cutting-edge technologies are uncertain, and another technology for mitigation of operating restriction or life extension of aging plants may be preferable, depending on the situation after unbundling and electricity market liberalization by the “Electricity System Reform” in a few years.
This is a preview of subscription content, log in via an institution to check access.
Access this chapter
- Available as PDF
- Read on any device
- Instant download
- Own it forever
- Available as EPUB and PDF
- Compact, lightweight edition
- Dispatched in 3 to 5 business days
- Free shipping worldwide - see info
- Durable hardcover edition
Tax calculation will be finalised at checkout
Purchases are for personal use only
Institutional subscriptions
Ministry of Economy, Trade and Industry (METI) (2012) Interim report on research council for the master plan of intensifying inter-regional tie-lines (Chiikikan rennkeisen tou no kyoka ni kansuru master plan kenkyukai chukan hokoku). METI, Tokyo, http://www.meti.go.jp/committee/sougouenergy/sougou/chiikikanrenkeisen/report01.html
Google Scholar
Ministry of Economy, Trade and Industry (METI) (2012) Summary of power supply and demand in FY 2011 (Heisei 23 nenndo denryoku jukyu no gaiyo). METI, Tokyo
Agency for Natural Resources and Energy (ANRE), Ministry of Economy, Trade and Industry (METI). Open database for statistical survey of electric power (Denryoku chosa toukei). http://www.enecho.meti.go.jp/statistics/electric_power/ep002/ METI, Tokyo.
Yamakawa M, Kakiuchi M, Katayama J (2006) 50 Hz large-capacity water-cooled generator and hydrogen-cooled generator. Toshiba Rev 61(4):56–59
The Japan gas association (JGA) (2013) CO 2 emission coefficient of electric energy using evaluation for CO 2 reduction countermeasures (CO 2 sakugen taisaku no hyoka ni mochiiru denki no CO 2 haisyutu keisuu ni tsuite). JGA, Tokyo, http://www.gas.or.jp/kankyo/co2taisaku_20130221.pdf
The Federation of Electric Power Companies of Japan (FEPC) (2010) Handbook of electric power industry (FY2010). The Japan Electric Association, ISBN: 978-4889482317
The Federation of Electric Power Companies of Japan (FEPC) (2013) Hand book of electric power industry (FY2013). The Japan Electric Association, ISBN: 978-4889482706
The Thermal and Nuclear Power Engineering Society (TENPES) (2011) Directory of thermal and nuclear power plants (FY 2011) (Karyoku genshiryoku hatudensho setsubi yoran 23 nendo kaitei ban). TEMPES, Tokyo
International Energy Agency (IEA) (1998) International coal trade: the evolution of a global market. IEA, Paris. ISBN 978-9264156678
Ministry of Economy, Trade and Industry (METI) (2012) New guidelines for practice of thermal power purchase by bids (Atarashii karyoku denngen nyusatsu no unyou ni kakaru shishin). METI, Tokyo, http://www.meti.go.jp/press/2012/09/20120918003/20120918003-2.pdf
Koyama M et al (2014) Present status and points of discussion for future energy systems in Japan from the aspects of technology options. JCEJ 47(7):499–513
Article Google Scholar
Ministry of Economy, Trade and Industry (METI) (2014) Reference table of best available technologies for thermal power plants (BAT no sankohyo). METI, Tokyo, http://www.meti.go.jp/policy/safety_security/industrial_safety/sangyo/electric/files/bat_sankouhyou/bat_20140501.pdf
Ministry of Economy, Trade and Industry (METI) (2014) Electricity system reform. METI, Tokyo, http://www.meti.go.jp/english/policy/energy_environment/electricity_system_reform/index.html
Ministry of Economy, Trade and Industry (METI) (2014) Present status and issues for natural gas and supply infrastructure in Japan (Wagakuni no tennen gas oyobi sono kyokyukiban no genjo to kadai). METI, Tokyo, http://www.meti.go.jp/committee/sougouenergy/sougou/kiban_seibi/001_06_00.pdf
Kimura H et al (2011) Development of technologies for highly-efficient coal-fired thermal power plants. Hitachi Hyoron 93(8):526–531
Ministry of Economy, Trade and Industry (METI) (2013) Promotion of introducing high efficiency thermal power plants (Kokoritu karyoku hatsuden no donyu suishin ni tsuite). METI, Tokyo, http://www.enecho.meti.go.jp/committee/council/basic_policy_subcommittee/past/002/pdf/002_009.pdf
Ministry of Economy, Trade and Industry (METI) (2010) Summary of element technology development for A-USC thermal power plants (Senshin cho-cho rinkaiatsu karyoku hatsuden jitsuyoka yousogijutsu kaihatsu no gaiyo ni tsuite). METI, Tokyo, http://www.meti.go.jp/committee/summary/0001640/034_05_09.pdf
Nunokawa M (2013) Progress in NAKOSO 250 MW air-blown IGCC demonstration project. Proceedings of ICOPE-2013, JSME
Fujii J (2013) The summary of the OSAKI CoolGen project and a future plan. J Jpn Inst Energy 92:408–412
New Energy and Industrial Techology Development Organization (NEDO) (2006) 2B5 next-generation, high-efficiency integrated coal gasification electric power generating process (A-IGCC/A-IGFC) clean coal technologies in Japan 32. NEDO, Kawasaki
Ito E et al (2011) Key technologies for ultra high temperature gas turbine. Mitsubishi Juko Gihou 48(3):2–7
Kobayashi Y et al (2011) Extremely high efficiency power system – SOFC triple combined cycle system. Mitsubishi Juko Gihou 48(3):16–21
Goto J et al (2011) Highly-efficient and flexible gas turbine system AHAT. Hitachi Hyoron 93(8):532–535
Ministry of Economy, Trade and Industry (METI) (2014) Hydrogen-firing power generation (Suiso hatsuden ni tsuite). METI, Tokyo, http://www.meti.go.jp/committee/kenkyukai/energy/suiso_nenryodenchi/suiso_nenryodenchi_wg/pdf/004_02_00.pdf
Imamura E, Nagano K (2010) Evaluation of life cycle CO 2 emissions of power generation technologies -update for state-of-the-art plants-, CRIEPI Report No. Y09027
Matsukata M, Koyama M (2012) Sweeping review of energy system in Japan (Zero kara minaosu energy) Maruzen, Japan ISBN: 978-4621085134
Download references
Author information
Authors and affiliations.
Waseda University, Tokyo, Japan
Takao Nakagaki
You can also search for this author in PubMed Google Scholar
Corresponding author
Correspondence to Takao Nakagaki .
Editor information
Editors and affiliations.
Tokyo Institute of Technology, Tokyo, Japan
Yukitaka Kato
Kyushu University, Fukuoka, Japan
Michihisa Koyama
Tohoku University, Miyagi, Japan
Yasuhiro Fukushima
Waseda University, Shinjuku-ku, Tokyo, Japan
Rights and permissions
Reprints and permissions
Copyright information
© 2016 Springer Japan
About this chapter
Nakagaki, T. (2016). Thermal Power Generation. In: Kato, Y., Koyama, M., Fukushima, Y., Nakagaki, T. (eds) Energy Technology Roadmaps of Japan. Springer, Tokyo. https://doi.org/10.1007/978-4-431-55951-1_14
Download citation
DOI : https://doi.org/10.1007/978-4-431-55951-1_14
Published : 31 May 2016
Publisher Name : Springer, Tokyo
Print ISBN : 978-4-431-55949-8
Online ISBN : 978-4-431-55951-1
eBook Packages : Energy Energy (R0)
Share this chapter
Anyone you share the following link with will be able to read this content:
Sorry, a shareable link is not currently available for this article.
Provided by the Springer Nature SharedIt content-sharing initiative
- Publish with us
Policies and ethics
- Find a journal
- Track your research
Research on Optimizing Heat Supply of Thermal Power Plants
Ieee account.
- Change Username/Password
- Update Address
Purchase Details
- Payment Options
- Order History
- View Purchased Documents
Profile Information
- Communications Preferences
- Profession and Education
- Technical Interests
- US & Canada: +1 800 678 4333
- Worldwide: +1 732 981 0060
- Contact & Support
- About IEEE Xplore
- Accessibility
- Terms of Use
- Nondiscrimination Policy
- Privacy & Opting Out of Cookies
A not-for-profit organization, IEEE is the world's largest technical professional organization dedicated to advancing technology for the benefit of humanity. © Copyright 2024 IEEE - All rights reserved. Use of this web site signifies your agreement to the terms and conditions.
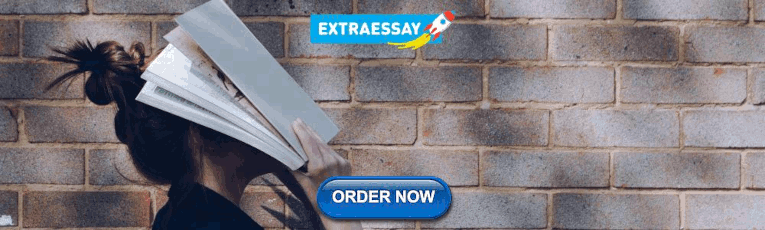
IMAGES
VIDEO
COMMENTS
A thermal power plant is a facility where heat energy is converted into electrical energy. This is achieved by transforming heat or steam into mechanical energy, which is then utilized to generate electricity. b. Energy Conversion Process: Most thermal power plants rely on steam or heat as the initial source of energy. This heat energy
The data of the power plant were obtained for a period of 8 years (2010-2017) from CEGCO annual reports 20,21.Throughout the studied period, several major malfunctions occurred to the power plant.
Fig. 3 showed an increase of 2.9% in global energy demand in 2018. This is the strongest growth since the year 2010, almost doubling the 10-year average. There was a significant increase in the demand for all sources of energy; however, gas witnesses the strongest increase in demand, presenting an estimated demand of 168 million tons (about 43 % of the total increase in global demand).
View PDF; Download full issue; Search ScienceDirect. Applied Thermal Engineering. Volume 240, 1 March 2024, 122231. Research Paper. Improving flexibility of thermal power plant through control strategy optimization based on orderly utilization of energy storage ... and it can be invoked for enhancing power ramp rate of thermal power plant ...
Plant efficiency is calculated when these sophisticated controllers are used. NLC Tamilnadu Power Ltd (NTPL), a 500 MW capacity plant has operating efficiency of 37.2% is given in Table 2.From Table 2, Load variation is achieved in NTPL plant in the order of 5 MW.As per the plant design, time to achieve 5 MW change was found to be 60sec when the conventional controller was used.
in an effort to improve the efficiency of thermal power plant [4]. In an A-USC thermal power plant, the steam parameters can reach 700⁰C-760⁰C, 30MPa-35MPa. The thermal efficiency of such a power plant can reach 55% (based on Lower Heating Value), where the major challenge lies in limitations of materials [5]. 3.2 Lowering final steam ...
This paper presents a critical review of the research conducted for modeling and controlling supercritical power plants. Thermal power plants are classified according to the boiler pressure to ...
Thermal power plants constitute the largest proportion of installed capability in global power generation system, which consumes large quantities of coal. Therefore achieving optimal operation of thermal power units, improving its efficiency and reducing the coal consumption is of great significance for the reduction of greenhouse gas and pollutants emissions. Modelling and simulation is the ...
Thermal Power Plant is converter of foss il fuel energy to. electricity in which during a cycle, steam is u sed to spin. a turbine driving electrical generator to p roduce. electricity. The first ...
The rmal energy is the major source of power generation The thermal. power plant de signed here works on the Reheat Rankine Cycle. It is a coal-based power plant. The. conversion from coal to ...
The systems and equipments in the thermal power plant should be operated with optimum efficiency. An assessment of these system performance and comparing it with the design value is necessary for its improvement. This paper presents the findings of the observations and trials carried out on thermal power plant energy equipments. The main ...
This paper presents the recent research on the study of the strategies for the flexible operation of the thermal power plant to meet the requirement of load balance. The study aimed to investigate the feasibility of bringing the High Temperature Thermal Energy Storage (HTTES) to the thermal power plant steam-water cycle, to identify the suitable HTTES in the cold (hot) section of the reheating ...
It was found that the flash tank enhanced the net power by 0.72%. Further, as a resultant of using flash tank, the energy efficiency of the system increased from 31.68% to 31.91% while saving 25444.47 cubic meters water per year. In terms of exergy efficiency, a 0.72% increase was achieved.
CO2-Tower. The CO2-Tower is a solar tower power plant with a steam turbine, a pressurized gas receiver and a pressurized solid media thermal energy storage. Fig. 1d shows the flow schematic of this system. CO2 is used as HTF, which is heated up in the cavity receiver with metal tubes on top of a tower from 310-600°C.
The chapter compares the exergetic performance of various biomass-fueled power plants, including combustion and gasification systems. It explores exergy analyses of biofuels use in internal combustion engines and gas turbines, polygeneration of electricity, heat, and chemicals, and biomass boilers and heating systems.
As a result, this impedes further improvement of the thermal efficiency of double reheat power plants. This paper presents two superheat utilization schemes of extraction steam in a double reheat ...
The energy efficiency of the plant is found to be 38.5%, 37.54% and 34.63% respectively for the plant operation at 250MW, 200MW and 125MW. A similar trend is seen in exergetic performance and exergy efficiency is recorded as 36.32%, 34.41% and 32.67% respectively at three loads mentioned above.
2.1 Overview of Thermal Power Generation Before and After Earthquake Disaster. After the earthquake disaster and subsequent nuclear power plant failure, the status of thermal power plants drastically changed. A summary of commercial thermal power generation operated by ten electric power companies and J-POWER in FY 2010 and 2014 is shown in Figs. 1a, b and 2a, b, respectively.
for capacity addition [1]. In the past period of time the power plant efficiency saw a surge from around 5-45%. The growing cognizance about the greenhouse gases further encourages the efficiency enhancement in the thermal power plants [2]. The efficiency of the power plant gradually deteriorates over a period of time. Retrofits can significantly
This paper presents emissions of carbon dioxide (CO 2), sulfur dioxide (SO 2), and nitric oxide (NO) from thermal power plants in India for a period of nine years from 2001-02 to 2009-10.
View PDF; Download full issue; Search ScienceDirect. Journal of Energy Storage. Volume 50, June 2022, 104335. Research Paper. Design and performance evaluation of a new thermal energy storage system integrated within a coal-fired power plant. ... For thermal power plants, the minimum power load ratio, a key aspect of operational flexibility, is ...
The heat supply mode of thermal power plants generally adopts central heating, which uses the high thermal energy generated by the complete combustion of coal for power generation, and the low thermal energy for heating, so as to realize the efficient utilization of energy. This paper expounds the present situation and existing problems of central heating in thermal power plants. This paper ...
Future CSP researchers will benefit from this paper's thorough overview of the technology, its potential prospect, and its research status. ... Real-time thermal power plants can incorporate such batteries. ... Before 2010, TES systems in power plants were primarily used for research into heat and mass transfer properties and validation of ...