Clinical Trials
Autism spectrum disorder.
Displaying 7 studies
The purpose of this study is to pilot test the ENHANCE intervention to evaluate methodology, feasibility based on caregiver input, and preliminary caregiver satisfaction. The primary outcomes will be feedback from parents, participant completion/atrition rates, the interventionist study log, and interventionist/caregiver/fidelity. Second, we aim to examine preliminary impact of the naturalistic intervention on child social communication and repetitive behavior in order to estimate clinically meaningful change for future research studies.
The purpose of this study is to examine a pilot intervention that will target social communication, cognition, and mood regulation. This multi-faceted, behavioral intervention can be individualized to improve independence and QoL in adults with Autism Spectrum Disorder (ASD) across the lifespan.
The purpose of this study is to confirm a correlation between metal ion dyshomeostasis (low Zn levels, abnormal Zn/Cu ratio, or low Selenium levels) in a North American population and the diagnosis of ASD using gold standard diagnostic instruments.
The purpose of this study is to determine whether a group recreation intervention for six to eight year old children with autism, who have deficits in gross motor skills is feasible and acceptable. Additionally the study will explore whether a group recreation intervention can improve gross motor skills, social skills, physical activity, and reduce parent stress.
Autism Spectrum Disorders (ASDs) are lifelong neurodevelopmental conditions with mostly unknown etiology that have a huge impact on affected individuals and their families, and are a major public health concern. Significant gaps exist in the knowledge of ASD frequency and its possible rising trend. Detailed characterization of ASD cases ASD subtypes is sparse, and potential risk factors (etiologies) are still unknown. We will study certain risk factors like perinatal risk factors and family history (parents, siblings) of psychiatric disorders. Knowledge about adult outcomes of ASD cases identified in childhood is almost non-existent. ASD-associated medical costs are high, yet information to ...
The purpose of this study is to screen mothers of young children with Autism Spectrum Disorders for metabolic problems, and find if it can be treated with a vitamin/mineral supplement with a different form of folic acid and more B12. These mothers will also be compared to the same number of mothers of normal children for the same metabolic markers.
This is an interview study to understand the views of people with the lived experience of 10 different genetic conditions on gene modification therapies, and specifically on prenatal gene editing. Prenatal gene editing is not happening now, but it is possible that prenatal gene editing will be available in the next few years, at least in a research setting, and we want to know your thoughts about the direction this technology is going. We to hope speak with many different stakeholders (patients and their families, clinicians, scientists) with diverse perspectives to understand values and priorities for prenatal gene ...
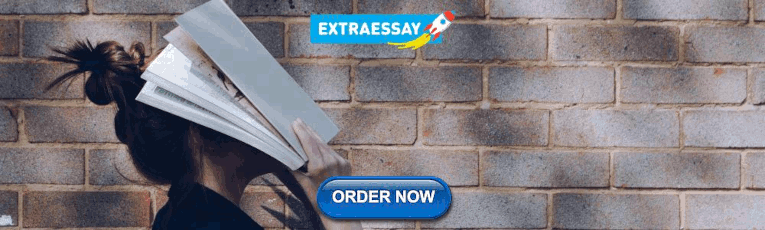
Mayo Clinic Footer
- Request Appointment
- About Mayo Clinic
- About This Site
Legal Conditions and Terms
- Terms and Conditions
- Privacy Policy
- Notice of Privacy Practices
- Notice of Nondiscrimination
- Manage Cookies
Advertising
Mayo Clinic is a nonprofit organization and proceeds from Web advertising help support our mission. Mayo Clinic does not endorse any of the third party products and services advertised.
- Advertising and sponsorship policy
- Advertising and sponsorship opportunities
Reprint Permissions
A single copy of these materials may be reprinted for noncommercial personal use only. "Mayo," "Mayo Clinic," "MayoClinic.org," "Mayo Clinic Healthy Living," and the triple-shield Mayo Clinic logo are trademarks of Mayo Foundation for Medical Education and Research.
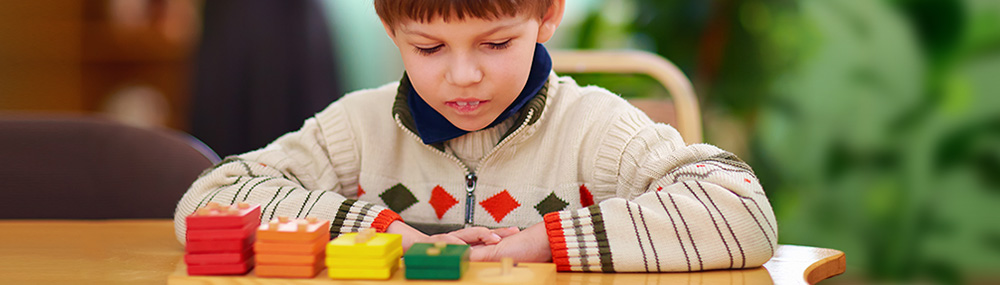
- Copy/Paste Link Link Copied
Find a Study on Autism
Select one of the following links to get ClinicalTrials.gov search results for studies on autism spectrum disorder (ASD):
- All NICHD clinical trials on ASD
- All ClinicalTrials.gov trials on ASD
Thank you for visiting nature.com. You are using a browser version with limited support for CSS. To obtain the best experience, we recommend you use a more up to date browser (or turn off compatibility mode in Internet Explorer). In the meantime, to ensure continued support, we are displaying the site without styles and JavaScript.
- View all journals
- My Account Login
- Explore content
- About the journal
- Publish with us
- Sign up for alerts
- Systematic Review
- Open access
- Published: 15 June 2022
Genetics of autism spectrum disorder: an umbrella review of systematic reviews and meta-analyses
- Shuang Qiu 1 ,
- Yingjia Qiu 2 ,
- Yan Li 3 &
- Xianling Cong ORCID: orcid.org/0000-0002-5790-4188 1
Translational Psychiatry volume 12 , Article number: 249 ( 2022 ) Cite this article
16k Accesses
21 Citations
9 Altmetric
Metrics details
- Autism spectrum disorders
Autism spectrum disorder (ASD) is a class of neurodevelopmental conditions with a large epidemiological and societal impact worldwide. To date, numerous studies have investigated the associations between genetic variants and ASD risk. To provide a robust synthesis of published evidence of candidate gene studies for ASD, we performed an umbrella review (UR) of meta-analyses of genetic studies for ASD (PROSPERO registration number: CRD42021221868). We systematically searched eight English and Chinese databases from inception to March 31, 2022. Reviewing of eligibility, data extraction, and quality assessment were performed by two authors. In total, 28 of 5062 retrieved articles were analyzed, which investigated a combined 41 single nucleotide polymorphisms (SNPs) of nine candidate genes. Overall, 12 significant SNPs of CNTNAP2 , MTHFR , OXTR , SLC25A12 , and VDR were identified, of which associations with suggestive evidence included the C677T polymorphism of MTHFR (under allelic, dominant, and heterozygote models) and the rs731236 polymorphism of VDR (under allelic and homozygote models). Associations with weak evidence included the rs2710102 polymorphism of CNTNAP2 (under allelic, homozygote, and recessive models), the rs7794745 polymorphism of CNTNAP2 (under dominant and heterozygote models), the C677T polymorphism of MTHFR (under homozygote model), and the rs731236 polymorphism of VDR (under dominant and recessive models). Our UR summarizes research evidence on the genetics of ASD and provides a broad and detailed overview of risk genes for ASD. The rs2710102 and rs7794745 polymorphisms of CNTNAP2 , C677T polymorphism of MTHFR , and rs731236 polymorphism of VDR may confer ASD risks. This study will provide clinicians and healthcare decision-makers with evidence-based information about the most salient candidate genes relevant to ASD and recommendations for future treatment, prevention, and research.
Similar content being viewed by others
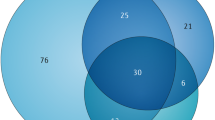
A framework for an evidence-based gene list relevant to autism spectrum disorder
Christian P. Schaaf, Catalina Betancur, … Jacob A. S. Vorstman
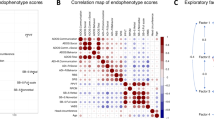
Quantitative trait locus analysis for endophenotypes reveals genetic substrates of core symptom domains and neurocognitive function in autism spectrum disorder
In-Hee Lee, Ekaterina Koelliker & Sek Won Kong
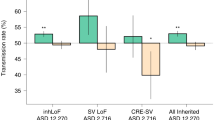
A phenotypic spectrum of autism is attributable to the combined effects of rare variants, polygenic risk and sex
Danny Antaki, James Guevara, … Jonathan Sebat
Introduction
Autism spectrum disorder (ASD) is a group of neurodevelopmental conditions characterized by early-onset dysfunctions in communication, impairments in social interaction, and repetitive and stereotyped behaviors and interests [ 1 ]. Patients develop ASD-related symptoms when they are 12−18 months of age, and diagnosis is generally made at the age of 2 years [ 2 ]. In 2010, 52 million people had been diagnosed with ASD worldwide, which was equivalent to a population prevalence of 7.6 per 1000 or 1 in 132 persons [ 3 ]. ASD is the leading cause of disability in children under 5 years, and people with ASD may require high levels of support, which is costly and thus leads to substantial economic, emotional, and physical burdens on affected families [ 3 ].
Due to the lack of clinical and epidemiological evidence for an ASD cure, researchers have focused on better understanding ASD and advancing risk prediction and prevention [ 3 ]. The causes of ASD are complex and multifactorial, with several associated genes and environmental risk factors [ 4 ]. A previous umbrella review (UR) of environmental risk factors for ASD showed that several maternal factors, including advanced age (≥35 years), chronic hypertension, preeclampsia, gestational hypertension, and being overweight before or during pregnancy, were significantly associated with ASD risk, without any signs of bias [ 5 , 6 ]. Accumulating twin- and family based studies further indicate that genetic factors play critical roles in ASD, such that the concordance rate among monozygotic twins is higher (60–90%) than that among dizygotic twins (0–30%) [ 7 , 8 ]. The heritability of ASD has been estimated to be 50%, indicating that genetic factors are the main contributors to the etiology of ASD [ 8 ].
To date, numerous studies investigating the association between genetic variants and ASD risk have been published [ 9 , 10 , 11 ]. Most of these studies focused on identifying single nucleotide polymorphisms (SNPs) of candidate genes associated with ASD risk. However, these SNP studies had small sample sizes and, therefore, low statistical power to demonstrate statistically significant effects of low-risk susceptibility genes, leading to inconsistent conclusions. Although meta-analyses have been conducted to resolve this problem, single SNPs or genes have usually been investigated.
An UR collects and evaluates multiple systematic reviews and meta-analyses conducted on a specific research topic, provides a robust synthesis of published evidence, and considers the importance of effects found over time [ 12 ]. In addition, the results of UR studies may increase the predictive power with more precise estimates [ 13 ]. Thus, we aimed to perform an UR study of all the systematic reviews and meta-analyses that have been published, assessing candidate genes associated with ASD risk. This study will provide clinicians and healthcare decision-makers with evidence-based information about candidate genes of ASD and recommendations for future prevention and research in less time than would otherwise be required to locate and examine all relevant research individually.
Literature search strategy and eligibility criteria
We systematically searched the PubMed, EMBASE, PsycINFO, Web of Science, Cochrane Library, China National Knowledge Infrastructure, Sinomed, and Wanfang databases from inception to March 31, 2022. The databases were searched using the following strategy: (autis* [All Fields] OR autism* [All Fields] OR autistic* [All Fields] OR ASD [All Fields] OR autism spectrum disorder* [All Fields] OR PDD-NOS [All Fields] OR PDDNOS [All Fields] OR unspecified PDD [All Fields] OR PDD [All Fields] OR pervasive developmental disorder* [All Fields] OR pervasive developmental disorder not otherwise specified [All Fields] OR Asperger* [All Fields] OR Asperger* syndrome [All Fields]) AND (gene* [All Fields] OR genom* [All Fields]) AND (systematic review [All Fields] OR meta-analysis [All Fields]). Authors S. Qiu and Y. Qiu independently conducted literature searches for potential articles included in this review. The references of the relevant articles were manually searched to identify and incorporate eligible studies.
We included meta-analyses of family based and case-control studies that examined associations between ASD and potential risk genes. We only included meta-analyses that reported either effect estimates of individual study or the data necessary to calculate these estimates. We excluded meta-analyses if (1) risk genes were used for screening, diagnostic, or prognostic purposes; (2) a study examined ASD as a risk factor for other medical conditions; (3) a study included fewer than three original studies investigating the association between risk genes and ASD; and (4) a study with missing information after the corresponding author, whom we contacted through email, failed to provide the required information. All articles retrieved were first organized in the reference manager software (Endnote 9, Clarivate Analytics, New York, NY, USA), and duplicates were deleted. S. Qiu and Y. Qiu chose eligible articles by screening the titles, abstracts, and full article texts independently. Disagreements were resolved through a discussion with a third investigator (Y. Li) until a consensus was reached.
Data extraction and quality assessment
From each eligible meta-analysis, we extracted the first author, publication year, genetic risk factors examined, number of studies, number of ASD cases and participants, study-specific relative risk estimates (odds ratio [ OR ]) with the corresponding 95% confidence interval ( CI ), sample size of cases and controls, genotype and allele counts, and individual study designs (case-control, family based or mixed [case-control and family based]). We used the ‘assessment of multiple systematic reviews’ tool, consisting of 11 items, to assess the methodological quality of the meta-analyses [ 14 ]. Data extraction and quality assessment were independently conducted by S. Qiu and Y. Qiu. Disagreements were resolved via a discussion with a third investigator (Y. Li) until a consensus was reached.
Data analysis
In agreement with previous URs, we performed a statistical analysis using a series of tests that were previously developed and reproduced [ 13 , 15 , 16 ]. If more than one meta-analysis on the same research question was eligible, the most recent meta-analysis was retained for the main analysis. For each eligible meta-analysis, we calculated the summary-effect size with 95% CI [ 17 ]. We also calculated the 95% prediction interval ( PI ) to explain the between-study heterogeneity and to assess the uncertainty of a new study [ 18 , 19 ]. Heterogeneity between studies was assessed using the Chi-squared test based Q-statistic and quantified using the I 2 -statistic [ 20 , 21 ]. If there was no substantial statistical heterogeneity ( P > 0.10, I 2 ≤ 50%), data were pooled using a fixed-effect model; otherwise, heterogeneity was evaluated using a random-effect model [ 22 ]. The Hardy–Weinberg equilibrium (HWE) of meta-analyses in the control group was analyzed using Chi-squared tests. Additionally, small-study effects were evaluated using Egger’s regression asymmetry test. P -values < 0.10 were considered to indicate the presence of small-study effects [ 23 , 24 ]. The Chi-squared test was used to assess the presence of excess significance, which evaluated whether the observed number of studies with significant results ( P < 0.05) was greater than the expected number [ 22 , 25 ]. All statistical analyses were performed using RStudio 3.6.2. Statistical significance was set at P < 0.05, except where otherwise specified.
Determining the credibility of evidence
In line with previous URs, we categorized the strength of the evidence of risk genes for ASD into five levels: convincing (class I), highly suggestive (class II), suggestive (class III), weak (class IV), and not significant [ 5 , 26 , 27 , 28 ]. Criteria for the level of evidence included the number of ASD cases, P -values by random effects model, small-study effects, excess significance bias, heterogeneity ( I² ), and 95% CI .
This review was prospectively registered with PROSPERO (registration number: CRD42021221868).
Description of eligible meta-analyses
A total of 5062 articles were identified through an initial search. After removing duplicates, the titles and abstracts of 3182 articles were screened for eligibility. Of the remaining 66 articles that were reviewed in full, 28 eligible articles were selected for data extraction (Fig. 1 ).
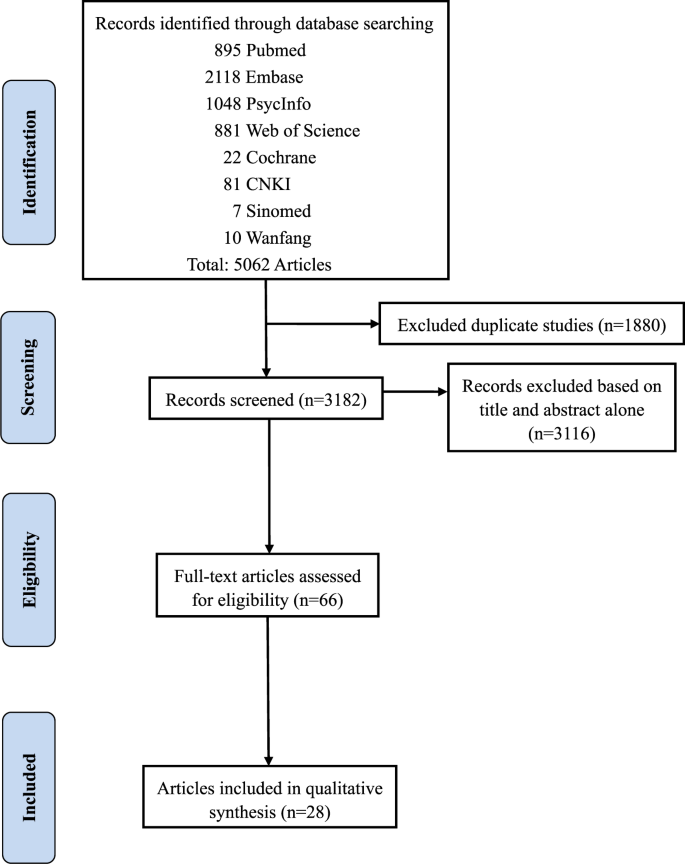
Flow chart of literature identification and selection.
The characteristics of the selected studies are presented in Table 1 . Of the 28 included reviews, eight were on methylenetetrahydrofolate reductase ( MTHFR ) [ 29 , 30 , 31 , 32 , 33 , 34 , 35 , 36 ]; four each on solute carrier family 6 member 4 ( SLC6A4 ) [ 37 , 38 , 39 , 40 ] and contactin associated protein 2 ( CNTNAP2 ) [ 41 , 42 , 43 , 44 ]; three each on oxytocin receptor ( OXTR ) [ 45 , 46 , 47 ] and reelin ( RELN ) [ 48 , 49 , 50 ]; two each on gamma-aminobutyric acid type A receptor subunit beta3 ( GABRB3 ) [ 51 , 52 ], solute carrier family 25 member 12 ( SLC25A12 ) [ 53 , 54 ], and vitamin D receptor ( VDR ) [ 55 , 56 ]; and one on catechol-o-methyltransferase ( COMT ) [ 39 ] (one meta-analysis was on both COMT and SLC6A4 ). These studies were published from 2008 to 2021 and considered the associations between 41 SNPs in nine candidate genes and ASD risk. For quality assessment, 22 articles that scored 5−8 were rated as ‘moderate quality’, and six that scored < 5 were rated as ‘low quality’. Seventeen studies (60.7%) performed the HWE check (Table 1 ). With respect to the study design, 14 (64.3%) studies synthesized case-control studies, two (7.1%) included family based studies, and eight (28.6%) used both case-control and family based studies (Table 1 ).
Summary-effect sizes and significant findings
The results of the associations between the 41 SNPs and ASD risks reported in the meta-analyses are presented in Table 2 under five different genetic models: allelic model (mutant allele vs. wild-type allele), dominant model (mutant homozygote + heterozygote vs. wild-type homozygote), heterozygote model (heterozygote vs. wild-type homozygote), homozygote model (mutant homozygote vs. wild-type homozygote), and recessive model (mutant homozygote vs. wild-type homozygote + heterozygote).
Only one meta-analysis on the rs2710102 polymorphism of CNTNAP2 showed that the polymorphism was associated with ASD susceptibility in allelic, homozygote, and recessive models [ 44 ]. This meta-analysis also found that the rs7794745 polymorphism of CNTNAP2 was associated with an increased risk of ASD in dominant and heterozygote models [ 44 ].
All four meta-analyses reported no significant association between the A1298C polymorphism of MTHFR and ASD risk. All eight meta-analyses on the C677T polymorphism of MTHFR showed that the polymorphism was associated with ASD susceptibility in allelic and heterozygote models [ 29 , 30 , 31 , 32 , 33 , 34 , 35 , 36 ]. Seven meta-analyses found that the C677T polymorphism was associated with an increased risk of ASD in dominant [ 29 , 31 , 32 , 33 , 34 , 35 , 36 ] and homozygote [ 29 , 30 , 31 , 33 , 34 , 35 , 36 ] models. Five meta-analyses found that the C677T polymorphism was associated with an increased risk of ASD in the recessive model [ 29 , 30 , 31 , 33 , 34 ].
For OXTR , 19 SNPs were summarized. LoParo et al. [ 45 ] found that the mutant allele of rs2268491, wild-type allele of rs237887, and mutant allele of rs7632287 were risk-inducing SNPs of ASD. In addition, Kranz et al. [ 46 ] found that the mutant allele of rs237889 was associated with ASD risk.
Regarding SLC25A12 , both Aoki et al. [ 53 ] and Liu et al. [ 54 ] found that the mutant alleles of rs2056202 and rs2292813 significantly increased ASD risk in family-based and mixed studies. We excluded the results of the associations between rs2292813 and ASD risk based on the case-control design reported by Liu et al. [ 54 ], as the authors included only two case–control studies.
Sun et al. [ 55 ] found that the rs2228570 polymorphism of VDR was associated with an increased ASD risk in homozygote and recessive models, while Yang et al. [ 56 ] did not find significant associations in any genetic model. Both authors [ 55 , 56 ] found that the rs731236 polymorphism of VDR was significantly associated with ASD risk in allelic, homozygote, and recessive models. Sun et al. [ 55 ] found that the rs731236 polymorphism was significantly associated with ASD risk in the dominant model. Both Sun et al. [ 55 ] and Yang et al. [ 56 ] found that the mutant allele of rs7975232 of VDR was significantly associated with a decreased ASD risk (Table 2 ). There were no significant SNPs in COMT , GABRB3 , RELN , and SLC6A4 .
When more than one meta-analysis on the same research question was eligible, the most recent one was retained for the main analysis. After comparing the publication year and sample size of each meta-analysis, 11 meta-analyses were retained for further analysis, of which two each study were on RELN and MTHFR , and one each was on CNTNAP2 , COMT , GABRB3 , OXTR , SLC25A12 , SLC6A4 , and VDR . We extracted the allele and genotype frequencies of each SNP in case and control groups from the original research for further analysis. However, the allele and genotype frequencies of some SNPs in the compared groups could not be extracted from the original research that did not contain the information, and we could not obtain this information from the corresponding authors of the studies. Finally, we analyzed the data of 20 SNPs with allele frequencies in 10 meta-analyses from 117 original studies and 16 SNPs with genotype frequencies in eight meta-analyses from 101 original studies. Associations were measured using five different genetic models (Tables 3 , 4 ).
We found that the rs2710102 polymorphism of CNTNAP2 was associated with a decreased ASD risk in the allelic ( OR = 0.849, 95% CI = 0.734–0.981, P = 0.0263), homozygote ( OR = 0.668, 95% CI = 0.470–0.950, P = 0.0248), and recessive ( OR = 0.715, 95% CI = 0.563–0.909, P = 0.0062) models. In addition, we found that the mutant allele of rs7794745 ( CNTNAP2 ) increased ASD risk based on the dominant ( OR = 1.300, 95% CI = 1.109–1.523, P = 0.0012) and heterozygote ( OR = 1.275, 95% CI = 1.081–1.504, P = 0.0039) models. The C677T polymorphism of MTHFR was associated with an increased ASD risk in the allelic ( OR = 1.799, 95% CI = 1.303–2.483, P = 0.0004), dominant ( OR = 1.959, 95% CI = 1.402–2.738, P < 0.0001), heterozygote ( OR = 1.767, 95% CI = 1.343–2.330, P < 0.0001), and homozygote ( OR = 1.795, 95% CI = 1.158–2.782, P = 0.0089) models. The rs607755 polymorphism of RELN was associated with an increased ASD risk in the allelic ( OR = 1.316, 95% CI = 1.029–1.683, P = 0.0284), dominant ( OR = 1.520, 95% CI = 1.061–2.178, P = 0.0226), heterozygote ( OR = 1.483, 95% CI = 1.016–2.165, P = 0.0411), and homozygote ( OR = 1.816, 95% CI = 1.051–3.136, P = 0.0324) models. The rs731236 polymorphism of VDR was associated with an increased ASD risk in the allelic ( OR = 1.297, 95% CI = 1.125–1.494, P = 0.0003), dominant ( OR = 1.304, 95% CI = 1.082–1.571, P = 0.0053), homozygote ( OR = 1.741, 95% CI = 1.258–2.409, P = 0.0008), and recessive ( OR = 1.613, 95% CI = 1.187–2.190, P = 0.0022) models. In addition, we found that the mutant allele of rs7975232 ( VDR ) decreased ASD risk ( OR = 0.823, 95% CI = 0.681–0.993, P = 0.0425) based on the allelic model. There was no significant association between the other SNPs and ASD risk (all P > 0.05; Table 4 ).
As for the results of PI , the null value was excluded in only four SNPs of rs2710102 ( CNTNAP2 ) under the allelic, homozygote, and recessive models; rs7794745 ( CNTNAP2 ) under the heterozygote model; rs607755 ( RELN ) and rs731236 ( VDR ) under the allelic and homozygote models (Table 4 ). When evaluating small-study effects using Egger’s regression asymmetry test, evidence for statistically significant small-study effects in the meta-analyses was identified in some SNPs. Supporting evidence included a meta-analysis on A1298C ( MTHFR ) under the allelic, dominant, and heterozygote models; a meta-analysis on C677T ( MTHFR ) under the five genetic models; a meta-analysis on rs20317 ( GABRB3 ) under the dominant and heterozygote models; one each on rs736707 ( RELN ) and rs1544410 ( VDR ) under the recessive and allelic models, respectively; and three meta-analyses on rs607755 ( RELN ), 5-HTTLPR ( SLC6A4 ), and rs7975232 ( VDR ) under the heterozygote model ( P < 0.10).
Hints of excess-statistical-significance bias were observed in rs2710102 ( CNTNAP2 ) under the allelic, homozygote, and recessive models; rs4680 ( COMT ) under the allelic model; rs20317 ( GABRB3 ) under the heterozygote model; A1298C ( MTHFR ) under allelic, dominant, heterozygote, and recessive models; C677T ( MTHFR ) under homozygote and recessive models; rs736707 ( RELN ) under allelic, dominant, and homozygote models; 5-HTTLPR ( SLC6A4 ) under allelic and recessive models; rs11568820 ( VDR ) under the dominant model; and rs731236 ( VDR ) under the heterozygote model, with statistically significant ( P < 0.05) excess of positive studies (Table 4 ).
We categorized the strength of the evidence of 20 SNPs for ASD into five levels. According to the criteria for the level of evidence, for rs2710102 ( CNTNAP2 ), the P -value based on the random effects model was significant at P < 0.05 under allelic, homozygote, and recessive models. Between-study heterogeneity was not significant ( P > 0.10, I² < 50.0%), the 95% PI did not exclude the null value, and there was no excess significance bias ( P > 0.05) under the five genetic models. For rs7794745 ( CNTNAP2 ), the P -value based on the random effects model was significant at P < 0.05 under dominant and heterozygote models. For C677T ( MTHFR ), there was a total of 2147 ASD cases, which was > 1000, and the P -value based on the random effects model was significant at P < 10 –3 under allelic, dominant, and heterozygote models. Moreover, it was significant at P < 0.05 under the homozygote model. Between-study heterogeneity was large ( I² > 50.0%) under the five genetic models, the 95% PI did not exclude the null value under the five genetic models, and there was no excess significance bias ( P > 0.05) under allelic, dominant, and heterozygote models. For rs731236 ( VDR ), there was a total of 1088 ASD cases, which was >1000, the P -value based on the random effects model was significant at P < 10 –3 under allelic and homozygote models, and the P -value was significant at P < 0.05 under dominant and recessive models. Between-study heterogeneity was not significant ( P > 0.10, I² < 50.0%), the 95% PI excluded the null value, and there was no small-study effect ( P > 0.10) and excess significance bias ( P > 0.05) under the five genetic models (Table 4 ). Thus, the rs2710102 ( CNTNAP2 ) was graded as weak evidence (class IV) under allelic, homozygote, and recessive models; rs7794745 ( CNTNAP2 ) was graded as weak evidence (class IV) under dominant and heterozygote models; the C677T ( MTHFR ) was graded as suggestive evidence (class III) under allelic, dominant, and heterozygote models; C677T ( MTHFR ) was graded as weak evidence (class IV) under the homozygote model; VDR (rs731236) was graded as suggestive evidence (class III) under allelic and homozygote models; and VDR (rs731236) was graded as weak evidence (class IV) under dominant and recessive models.
This UR summarizes evidence on the genetic basis of ASD. Our study design provides a robust and significant synthesis of published evidence and increases the conclusive power with more precise estimates. Overall, 12 significant SNPs of CNTNAP2 , MTHFR , OXTR , SLC25A12 , and VDR were identified from 41 SNPs of nine candidate genes in 28 meta-analyses. Of those, associations with suggestive evidence (class III) were the C677T polymorphism of MTHFR (under allelic, dominant, and heterozygote models) and rs731236 polymorphism of VDR (under allelic and homozygote models). Associations with weak evidence (class IV) were the rs2710102 polymorphism of CNTNAP2 (under allelic, homozygote, and recessive models), rs7794745 polymorphism of CNTNAP2 (under dominant and heterozygote models), C677T polymorphism of MTHFR (under homozygote model), and rs731236 polymorphism of VDR (under dominant and recessive models).
ASD remains a ‘disease of theories’, as multiple genes and environmental risk factors are probably involved in its pathogenesis. However, to date, the etiology and pathological mechanism of ASD are still unknown [ 57 ]. The genetic architecture of ASD is complex. Moreover, most research in this field has focused on candidate genes, primarily those with a plausible role in the known underlying pathophysiology, including mitochondrial dysfunction, abnormal neurodevelopment, and dysfunction of synapse formation and stability during neurodevelopment [ 58 , 59 ].
CNTNAP2 is a member of neurexin superfamily and is a synaptic protein [ 60 ]. It plays a major role in neural development, crucial for neural circuit assembly [ 61 ]. CNTNAP2 mutations may be linked to the abnormal behavior of ASD by altering synaptic neurotransmission, functional connectivity, and neuronal network activity [ 61 , 62 ]. The rs2710102 and rs7794745 are two common non-coding variants in CNTNAP2 , with four and three meta-analyses reporting the associations with ASD, respectively. The results of the meta-analysis by Uddin et al. were inconsistent with the other authors’ [ 44 ]. We further re-analyzed and categorized the strengths of evidence. Both the rs2710102 and rs7794745 polymorphisms of CNTNAP2 were associated with decreased risk of ASD. The rs2710102 was graded as having a weak association with ASD under allelic, homozygote, and recessive models. The rs7794745 was graded as having a weak association with ASD under dominant and heterozygote models. Therefore, it is likely that the rs2710102 and rs7794745 polymorphisms of CNTNAP2 influence the risk of ASD.
MTHFR is one of the most frequently-researched genes in ASD, with four and eight meta-analyses for A1298C [ 29 , 31 , 32 , 33 ] and C667T [ 29 , 30 , 31 , 32 , 33 , 34 , 35 , 36 ] polymorphisms, respectively. The A1298C and C667T polymorphisms of MTHFR are associated with reduced enzymatic activity, which affects folate metabolism, and, consequently, fetal brain development [ 29 , 32 , 33 ]. Dysfunction of the brain is indicated in ASD etiology; thus, MTHFR has been the focal point of investigation in this disorder. The meta-analysis by Li et al. was selected because it was the most recent among the examined meta-analyses [ 34 ]. The genotype distributions of the A1298C and C667T polymorphisms of MTHFR in the control group were not found in the HWE, which may be due to selection bias, population stratification, and genotyping errors within the original studies. We found no significant association between the A1298C polymorphism of MTHFR and ASD risk in the five genetic models, which was consistent with the four meta-analyses, indicating that the A1298C polymorphism of MTHFR may not be a risk SNP of ASD. We found that the C667T polymorphism of MTHFR was associated with an increased risk of ASD, graded as having suggestive association under allelic, dominant, and heterozygote models and weak association under the homozygote model. Thus, the C667T polymorphism of MTHFR may confer ASD risk.
OXTR, a neuropeptide gene, is also one of the most frequently-studied genes associated with ASD [ 45 ]. Oxytocin plays an important role in a range of human behaviors, including affiliative behavior to social bonding, and is differentially expressed in the blood of individuals with autism compared to that of non-autistic individuals [ 45 , 63 ]. Three meta-analyses investigated 19 SNPs and ASD risk. Of these, only rs2254298 and rs53576 were analyzed in two meta-analyses [ 45 , 46 ], and the remaining SNPs were unique in one meta-analysis. Three SNPs (rs2268491, rs237887, and rs7632287) were significantly associated with ASD risk [ 45 , 46 ]; however, we failed to determine the credibility of the evidence because of the lack of original data.
RELN encodes a large secreted extracellular matrix protein considered to be involved in neuronal migration, brain structure construction, synapse formation, and stability during neurodevelopment [ 59 ]. Fatemi et al. found decreased levels of reelin mRNA and protein and increased levels of reelin receptors in the brain and plasma of individuals with autism [ 64 ]. Dysfunction of the reelin signaling pathway has been found in ASD, schizophrenia, epilepsy, bipolar disorder, mental retardation, depression, Alzheimer’s disease, and lissencephaly [ 59 , 65 ]. Genetic association studies have been conducted to investigate the associations between SNPs within RELN and ASD with conflicting results. None of the three meta-analyses found significant associations [ 48 , 49 , 50 ]. The meta-analysis by Hernández-García et al. was retained for further analysis of the original studies after comparing publication years and sample sizes of the three meta-analyses [ 50 ]. Hernández-García et al. did not find a significant association between RELN and ASD risk [ 50 ]. In our analysis, because there was no substantial statistical heterogeneity under the five genetic models (all P > 0.10, I 2 ≤ 50%), a fixed model was applied to pool the effect size. We found that the rs607755 of RELN was associated with ASD risk in allelic, dominant, heterozygote, and homozygote models. This inconsistent result was caused by different pooling methods, indicating that it is necessary to perform an UR to provide a robust synthesis of published evidence and evaluate the importance of genetic factors related to ASD. Our UR results showed that the rs607755 of RELN was not significant when we categorized the strength of the evidence. Thus, it may not be a risk factor for ASD.
SLC25A12 encodes the mitochondrial aspartate/glutamate carrier of the brain, a calcium-binding solute carrier located in the inner mitochondrial membrane that is expressed principally in the heart, brain, and skeletal muscle [ 66 , 67 ]. Rossignol et al. found that individuals with ASD had a significantly higher prevalence of mitochondrial diseases than that of controls, indicating the involvement of mitochondrial dysfunction in ASD [ 58 ]. Thus, an increasing number of genetic studies on ASD have focused on SLC25A12 . However, the results on the association between SNPs of SLC25A12 and ASD risk are inconsistent. Two meta-analyses were performed by Aoki et al. [ 53 ] and Liu et al. [ 54 ], and despite differences in the number of studies between the two meta-analyses, both found a higher risk of ASD in individuals with the mutant allele of rs2056202 or rs2292813. However, we failed to determine the credibility of the evidence because of a lack of original data.
Vitamin D plays a significant role in brain homeostasis, neurodevelopment, and immunological modulation, and its deficiency has been reported in children with ASD [ 68 ]. Hence, changes in the genes involved in the transport or binding of vitamin D may be associated with ASD risk. Notably, vitamin D exerts its effects on genes via the VDR gene, to which changes may be an underlying risk factor for ASD. Sun et al. [ 55 ] and Yang et al. [ 56 ] performed meta-analyses to pool the effect size of inconsistent conclusions from original studies on the associations between SNPs in VDR and ASD risks. We further re-analyzed and categorized the strengths of evidence. The rs731236 polymorphism of VDR was associated with an increased risk of ASD, graded as having a suggestive association under allelic and homozygote models and a weak association under dominant and recessive models without small-study effects, excess significance bias, and large heterogeneity. It is likely that the VDR rs731236 polymorphism influences the risk of ASD.
Our study has some limitations. First, associations between several SNPs and ASD risks under five genetic models or in different populations were not fully assessed in our UR, partly due to insufficient original data. Second, our UR is limited by significant heterogeneity that may be caused by population stratification, study design, and differences in the pattern of linkage disequilibrium structure. Finally, ASD is a complex disorder with different causative factors (multiple genetic and environmental factors). We did not investigate the involvement of environmental factors in ASD. Despite these limitations above, our UR includes its prospective registration with PROSPERO, an extensive search strategy, clear criteria of inclusion and exclusion, duplicated processing by two authors, accurate quality assessment, systematic assessment and critical comparison of meta-analyses, and consistent standards for re-analysis of original data.
In conclusion, our UR summarizes evidence on the genetics of ASD and provides a broad and detailed overview of risk genes for ASD. The rs2710102 and rs7794745 polymorphisms of CNTNAP2 , C677T polymorphism of MTHFR , and rs731236 polymorphism of VDR may confer ASD risk. This study will aid clinicians in decision-making through the use of evidence-based information on the most salient candidate genes relevant to ASD and recommendations for future treatment, prevention, and research.
Lai MC, Lombardo MV, Baron-Cohen S. Autism. Lancet. 2014;383:896–910.
Article PubMed Google Scholar
WHO Questions and answers about autism spectrum disorders (ASD). 2021; http://www.who.int/features/qa/85/en/ . Accessed 5 July 2021.
Baxter AJ, Brugha TS, Erskine HE, Scheurer RW, Vos T, Scott JG. The epidemiology and global burden of autism spectrum disorders. Psychological Med. 2015;45:601–13.
Article CAS Google Scholar
Chaste P, Leboyer M. Autism risk factors: genes, environment, and gene-environment interactions. Dialogues Clin Neurosci. 2012;14:281–92.
Article PubMed PubMed Central Google Scholar
Kim JY, Son MJ, Son CY, Radua J, Eisenhut M, Gressier F, et al. Environmental risk factors and biomarkers for autism spectrum disorder: an umbrella review of the evidence. lancet Psychiatry. 2019;6:590–600.
Lord C, Brugha TS, Charman T, Cusack J, Dumas G, Frazier T, et al. Autism spectrum disorder. Nat Rev Dis Prim. 2020;6:5.
Ronald A, Hoekstra RA. Autism spectrum disorders and autistic traits: a decade of new twin studies. Am J Med Genet Part B, Neuropsychiatr Genet: Off Publ Int Soc Psychiatr Genet. 2011;156b:255–74.
Article Google Scholar
Sandin S, Lichtenstein P, Kuja-Halkola R, Larsson H, Hultman CM, Reichenberg A. The familial risk of autism. JAMA. 2014;311:1770–7.
Article CAS PubMed PubMed Central Google Scholar
Peñagarikano O, Abrahams BS, Herman EI, Winden KD, Gdalyahu A, Dong H, et al. Absence of CNTNAP2 leads to epilepsy, neuronal migration abnormalities, and core autism-related deficits. Cell. 2011;147:235–46.
Article PubMed PubMed Central CAS Google Scholar
Qiu S, Li Y, Bai Y, Shi J, Cui H, Gu Y, et al. SHANK1 polymorphisms and SNP-SNP interactions among SHANK family: a possible cue for recognition to autism spectrum disorder in infant age. Autism Res. 2019;12:375–83.
Grove J, Ripke S, Als TD, Mattheisen M, Walters RK, Won H, et al. Identification of common genetic risk variants for autism spectrum disorder. Nat Genet. 2019;51:431–44.
Ioannidis JP. Integration of evidence from multiple meta-analyses: a primer on umbrella reviews, treatment networks and multiple treatments meta-analyses. CMAJ: Can Med Assoc J = J de l’Assoc Med Canadienne. 2009;181:488–93.
van der Burg NC, Al Hadithy AFY, van Harten PN, van Os J, Bakker PR. The genetics of drug-related movement disorders, an umbrella review of meta-analyses. Mol Psychiatry. 2020;25:2237–50.
Shea BJ, Hamel C, Wells GA, Bouter LM, Kristjansson E, Grimshaw J, et al. AMSTAR is a reliable and valid measurement tool to assess the methodological quality of systematic reviews. J Clin Epidemiol. 2009;62:1013–20.
Giannakou K, Evangelou E, Papatheodorou SI. Genetic and non-genetic risk factors for pre-eclampsia: umbrella review of systematic reviews and meta-analyses of observational studies. Ultrasound Obstet Gynecol. 2018;51:720–30.
Article CAS PubMed Google Scholar
Yang T, Li X, Montazeri Z, Little J, Farrington SM, Ioannidis JPA, et al. Gene-environment interactions and colorectal cancer risk: an umbrella review of systematic reviews and meta-analyses of observational studies. Int J Cancer. 2019;145:2315–29.
Lau J, Ioannidis JP, Schmid CH. Quantitative synthesis in systematic reviews. Ann Intern Med. 1997;127:820–6.
Higgins JP, Thompson SG, Spiegelhalter DJ. A re-evaluation of random-effects meta-analysis. J Roy Soc Statistical Soc A (Stat Soc). 2009;172:137–59.
Higgins JP. Commentary: Heterogeneity in meta-analysis should be expected and appropriately quantified. Int J Epidemiol. 2008;37:1158–60.
Cochran WGJB. Combination Estimates Differ Exp. 1954;10:101–29.
Google Scholar
Higgins JP, Thompson SG. Quantifying heterogeneity in a meta-analysis. Stat Med. 2002;21:1539–58.
Ioannidis JP, Patsopoulos NA, Evangelou E. Uncertainty in heterogeneity estimates in meta-analyses. BMJ (Clin Res Ed). 2007;335:914–6.
Sterne JA, Sutton AJ, Ioannidis JP, Terrin N, Jones DR, Lau J, et al. Recommendations for examining and interpreting funnel plot asymmetry in meta-analyses of randomised controlled trials. BMJ (Clin Res Ed). 2011;343:d4002.
Egger M, Davey Smith G, Schneider M, Minder C. Bias in meta-analysis detected by a simple, graphical test. Clin Focus. 1997;315:629–34.
CAS Google Scholar
Ioannidis JPA. Clarifications on the application and interpretation of the test for excess significance and its extensions. J Math Psychol. 2013;57:184–7.
Belbasis L, Köhler CA, Stefanis N, Stubbs B, van Os J, Vieta E, et al. Risk factors and peripheral biomarkers for schizophrenia spectrum disorders: an umbrella review of meta-analyses. Acta Psychiatr Scand. 2018;137:88–97.
Bellou V, Belbasis L, Tzoulaki I, Evangelou E, Ioannidis JP. Environmental risk factors and Parkinson’s disease: an umbrella review of meta-analyses. Parkinsonism Relat Disord. 2016;23:1–9.
Belbasis L, Bellou V, Evangelou E, Ioannidis JP, Tzoulaki I. Environmental risk factors and multiple sclerosis: an umbrella review of systematic reviews and meta-analyses. Lancet Neurol. 2015;14:263–73.
Pu D, Shen Y, Wu J. Association between MTHFR gene polymorphisms and the risk of autism spectrum disorders: a meta-analysis. Autism Res. 2013;6:384–92.
Rai V. Association of methylenetetrahydrofolate reductase (MTHFR) gene C677T polymorphism with autism: evidence of genetic susceptibility. Metab Brain Dis. 2016;31:727–35.
Sadeghiyeh T, Dastgheib SA, Mirzaee-Khoramabadi K, Morovati-Sharifabad M, Akbarian-Bafghi MJ, Poursharif Z, et al. Association of MTHFR 677C>T and 1298A>C polymorphisms with susceptibility to autism: a systematic review and meta-analysis. Asian J Psychiatry. 2019;46:54–61.
Razi B, Imani D, Hassanzadeh Makoui M, Rezaei R, Aslani S. Association between MTHFR gene polymorphism and susceptibility to autism spectrum disorders: systematic review and meta-analysis. Res Autism Spectrum Disorders. 2020;70:101473.
Li Y, Qiu S, Shi J, Guo Y, Li Z, Cheng Y, et al. Association between MTHFR C677T/A1298C and susceptibility to autism spectrum disorders: a meta-analysis. BMC Pediatrics. 2020;20:449.
Li CX, Liu YG, Che YP, Ou JL, Ruan WC, Yu YL, et al. Association between MTHFR C677T polymorphism and susceptibility to autism spectrum disorders: a meta-analysis in Chinese Han population. Front Pediatrics. 2021;9:598805.
Wang S, Wu J. Association between MTHFR gene C677T polymorphism and risk of autism spectrum disorder in children: a Meta-analysis. Chin J Obstet Gynecol Pediatr. 2021;17:198–206.
Zhang Y, Gai C, Yang L, Ma H, Zhang J, Sun H, et al. Meta-analysis of the relationship between MTHFR C677T gene polymorphism and susceptibility to autism spectrum disorders. Tianjin Med J. 2021;49:212–8.
Huang CH, Santangelo SL. Autism and serotonin transporter gene polymorphisms: a systematic review and meta-analysis. Am J Med Genet B: Neuropsychiatr Genet. 2008;147b:903–13.
Mo S, Qi X, Shao S, Sun Z, Song R. An integrated meta-analysis of the association between 5-HTTLPR and autism spectrum disorder. Acta Med Univ Sci Technol Huazhong. 2013;42:181–6.
Yang PY, Menga YJ, Li T, Huang Y. Associations of endocrine stress-related gene polymorphisms with risk of autism spectrum disorders: evidence from an integrated meta-analysis. Autism Res. 2017;10:1722–36.
Wang H, Yin F, Gao J, Fan X. Association between 5-HTTLPR polymorphism and the risk of autism: a meta-analysis based on case-control studies. Front psychiatry. 2019;10:51.
CAS PubMed PubMed Central Google Scholar
Werling AM, Bobrowski E, Taurines R, Gundelfinger R, Romanos M, Grünblatt E, et al. CNTNAP2 gene in high functioning autism: no association according to family and meta-analysis approaches. J Neural Transm. 2016;123:353–63. (Vienna, Austria: 1996)
Zhang T, Zhang J, Wang Z, Jia M, Lu T, Wang H, et al. Association between CNTNAP2 polymorphisms and autism: a family-based study in the chinese han population and a meta-analysis combined with GWAS data of psychiatric genomics consortium. Autism Res. 2019;12:553–61.
Wang Y, Liu Y, Xia Z, Yu H, Gai Z. Association of the contactin-association protein-like 2 gene rs2710102 polymorphism and autism spectrum disorders: a meta-analysis. Clin Focus. 2019;34:1010–4.
Uddin MS, Azima A, Aziz MA, Aka TD, Jafrin S, Millat MS, et al. CNTNAP2 gene polymorphisms in autism spectrum disorder and language impairment among Bangladeshi children: a case-control study combined with a meta-analysis. Hum Cell. 2021;34:1410–23.
LoParo D, Waldman ID. The oxytocin receptor gene (OXTR) is associated with autism spectrum disorder: a meta-analysis. Mol Psychiatry. 2015;20:640–6.
Kranz TM, Kopp M, Waltes R, Sachse M, Duketis E, Jarczok TA, et al. Meta-analysis and association of two common polymorphisms of the human oxytocin receptor gene in autism spectrum disorder. Autism Res. 2016;9:1036–45.
Zhou J. Association between the single nucleotide polymorphism (SNP) of oxytocin receptor (OXTR) gene and Autism Spectrum Disorders (ASD): a meta-analysis. Jining Medical University. 2020.
Wang Z, Hong Y, Zou L, Zhong R, Zhu B, Shen N, et al. Reelin gene variants and risk of autism spectrum disorders: an integrated meta-analysis. Am J Med Genet Part B: Neuropsychiatr Genet. 2014;165b:192–200.
Chen N, Bao Y, Xue Y, Sun Y, Hu D, Meng S, et al. Meta-analyses of RELN variants in neuropsychiatric disorders. Behav Brain Res. 2017;332:110–9.
Hernández-García I, Chamorro AJ, de la Vega HGT, Carbonell C, Marcos M, Mirón-Canelo JA. Association of allelic variants of the reelin gene with autistic spectrum disorder: A systematic review and meta-analysis of candidate gene association studies. Int J Environ Res Public Health. 2020;17:1–16.
Mahdavi M, Kheirollahi M, Riahi R, Khorvash F, Khorrami M, Mirsafaie M. Meta-analysis of the association between GABA receptor polymorphisms and autism spectrum disorder (ASD). J Mol Neurosci. 2018;65:1–9.
Noroozi R, Taheri M, Ghafouri-Fard S, Bidel Z, Omrani MD, Moghaddam AS, et al. Meta-analysis of GABRB3 gene polymorphisms and susceptibility to autism spectrum disorder. J Mol Neurosci. 2018;65:432–7.
Aoki Y, Cortese S. Mitochondrial aspartate/glutamate carrier SLC25A12 and autism spectrum disorder: a meta-analysis. Mol Neurobiol. 2016;53:1579–88.
Liu J, Yang A, Zhang Q, Yang G, Yang W, Lei H, et al. Association between genetic variants in SLC25A12 and risk of autism spectrum disorders: An integrated meta-analysis. Am J Med Genet Part B: Neuropsychiatr Genet. 2015;168b:236–46.
Sun J. Association between vitamin D receptor gene polymorphism and susceptibility to autism spectrum disorders: a meta-analysis. Jining Medical University. 2020.
Yang H, Wu X. The correlation between vitamin D receptor (VDR) gene polymorphisms and autism: a meta-analysis. J Mol Neurosci. 2020;70:260–8.
Kojic M, Gawda T, Gaik M, Begg A, Salerno-Kochan A, Kurniawan ND, et al. Elp2 mutations perturb the epitranscriptome and lead to a complex neurodevelopmental phenotype. Nat Commun. 2021;12:2678.
Rossignol DA, Frye RE. Mitochondrial dysfunction in autism spectrum disorders: a systematic review and meta-analysis. Mol psychiatry. 2012;17:290–314.
Jossin Y. Reelin functions, mechanisms of action and signaling pathways during brain development and maturation. Biomolecules. 2020;10:964.
Arking DE, Cutler DJ, Brune CW, Teslovich TM, West K, Ikeda M, et al. A common genetic variant in the neurexin superfamily member CNTNAP2 increases familial risk of autism. Am J Hum Genet. 2008;82:160–4.
Lazaro MT, Taxidis J, Shuman T, Bachmutsky I, Ikrar T, Santos R, et al. Reduced prefrontal synaptic connectivity and disturbed oscillatory population dynamics in the CNTNAP2 model of autism. Cell Rep. 2019;27:2567–2578.e2566.
Toma C, Pierce KD, Shaw AD, Heath A, Mitchell PB, Schofield PR, et al. Comprehensive cross-disorder analyses of CNTNAP2 suggest it is unlikely to be a primary risk gene for psychiatric disorders. PLoS Genet. 2018;14:e1007535.
Modahl C, Green L, Fein D, Morris M, Waterhouse L, Feinstein C, et al. Plasma oxytocin levels in autistic children. Biol psychiatry. 1998;43:270–7.
Fatemi SH. Reelin glycoprotein: structure, biology and roles in health and disease. Mol psychiatry. 2005;10:251–7.
Fatemi SH. Reelin glycoprotein in autism and schizophrenia. Int Rev Neurobiol. 2005;71:179–87.
Silverman JM, Buxbaum JD, Ramoz N, Schmeidler J, Reichenberg A, Hollander E, et al. Autism-related routines and rituals associated with a mitochondrial aspartate/glutamate carrier SLC25A12 polymorphism. Am J Med Genet B: Neuropsychiatr Genet. 2008;147:408–10.
Anitha A, Nakamura K, Thanseem I, Yamada K, Iwayama Y, Toyota T, et al. Brain region-specific altered expression and association of mitochondria-related genes in autism. Mol Autism. 2012;3:12.
Saad K, Abdel-Rahman AA, Elserogy YM, Al-Atram AA, Cannell JJ, Bjørklund G, et al. Vitamin D status in autism spectrum disorders and the efficacy of vitamin D supplementation in autistic children. Nutritional Neurosci. 2016;19:346–51.
Download references
Acknowledgements
This study was funded by the Science and Technology Department of Jilin Province (grant number: 20200601010JC).
Author information
Authors and affiliations.
Department of Biobank, China-Japan Union Hospital, Jilin University, Changchun, 130033, Jilin, China
Shuang Qiu & Xianling Cong
China-Japan Union Hospital, Jilin University, Changchun, 130033, Jilin, China
Yingjia Qiu
Department of Epidemiology, School of Public Health, Beihua University, Jilin, 132013, Jilin, China
You can also search for this author in PubMed Google Scholar
Contributions
Study design: S.Q. and X.C. Data collection, analysis, and interpretation: S.Q., Y.Q., and Y.L. Drafting of the manuscript: S.Q. Critical revision of the manuscript: X.C. Approval of the final version for publication: all co-authors.
Corresponding author
Correspondence to Xianling Cong .
Ethics declarations
Competing interests.
The authors declare no competing interests.
Additional information
Publisher’s note Springer Nature remains neutral with regard to jurisdictional claims in published maps and institutional affiliations.
Rights and permissions
Open Access This article is licensed under a Creative Commons Attribution 4.0 International License, which permits use, sharing, adaptation, distribution and reproduction in any medium or format, as long as you give appropriate credit to the original author(s) and the source, provide a link to the Creative Commons license, and indicate if changes were made. The images or other third party material in this article are included in the article’s Creative Commons license, unless indicated otherwise in a credit line to the material. If material is not included in the article’s Creative Commons license and your intended use is not permitted by statutory regulation or exceeds the permitted use, you will need to obtain permission directly from the copyright holder. To view a copy of this license, visit http://creativecommons.org/licenses/by/4.0/ .
Reprints and permissions
About this article
Cite this article.
Qiu, S., Qiu, Y., Li, Y. et al. Genetics of autism spectrum disorder: an umbrella review of systematic reviews and meta-analyses. Transl Psychiatry 12 , 249 (2022). https://doi.org/10.1038/s41398-022-02009-6
Download citation
Received : 08 January 2022
Revised : 22 May 2022
Accepted : 27 May 2022
Published : 15 June 2022
DOI : https://doi.org/10.1038/s41398-022-02009-6
Share this article
Anyone you share the following link with will be able to read this content:
Sorry, a shareable link is not currently available for this article.
Provided by the Springer Nature SharedIt content-sharing initiative
This article is cited by
Support vector machine prediction of individual autism diagnostic observation schedule (ados) scores based on neural responses during live eye-to-eye contact.
- J. Adam Noah
Scientific Reports (2024)
Parental Perspectives on Early Life Screening and Genetic Testing for ASD: A Systematic Review
- Katerina Dounavi
- Meral Koldas
Journal of Autism and Developmental Disorders (2024)
Altered behavior, brain structure, and neurometabolites in a rat model of autism-specific maternal autoantibody exposure
- Matthew R. Bruce
- Amalie C. M. Couch
- Judy Van de Water
Molecular Psychiatry (2023)
Calciopathies and Neuropsychiatric Disorders: Physiological and Genetic Aspects
- N. A. Dyuzhikova
- M. B. Pavlova
Neuroscience and Behavioral Physiology (2023)
Quick links
- Explore articles by subject
- Guide to authors
- Editorial policies

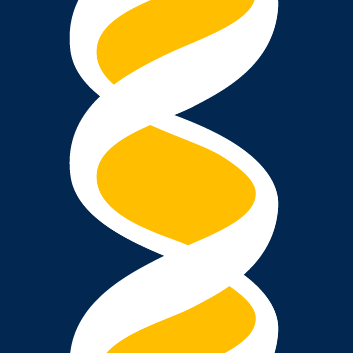
College of Biological Sciences
New research suggests cerebellum may play important role in autism.
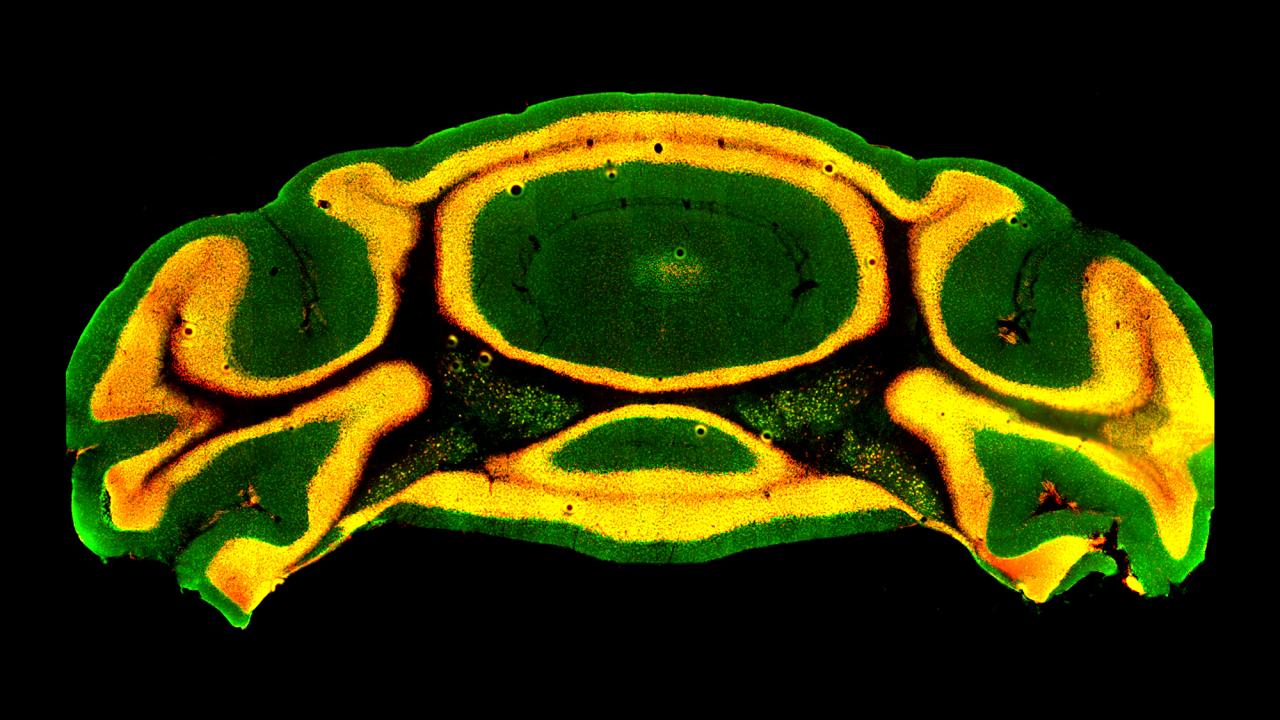
NIMH grant will fund studies on how autism risk gene impacts a crucial, but long-overlooked brain area
- by Douglas Fox
- April 02, 2024
Researchers in the College of Biological Sciences have received a grant to study the role of the cerebellum in autism. “ We need a more holistic understanding of the brain circuits that drive this disorder , ” says Alex Nord, an associate professor of neurobiology, physiology and behavior (NPB), and a researcher at the Center for Neuroscience (CNS). “ The cerebellum is a key component that has been largely overlooked until recently . ”
Nord partnered with Diasynou Fioravante, also an associate professor of NPB and CNS researcher, and received an R21 grant from the National Institute of Mental Health (NIMH). Announced in November, it will provide $435,000 of funding over the next two years.
With this grant, they will study how a potent autism risk gene, called chromodomain helicase DNA binding protein 8 ( Chd8 ), alters function in the cerebellum, which plays a crucial role in physical movement, and how this drives autism-like behaviors. Their research ties together two emerging trends in autism research.
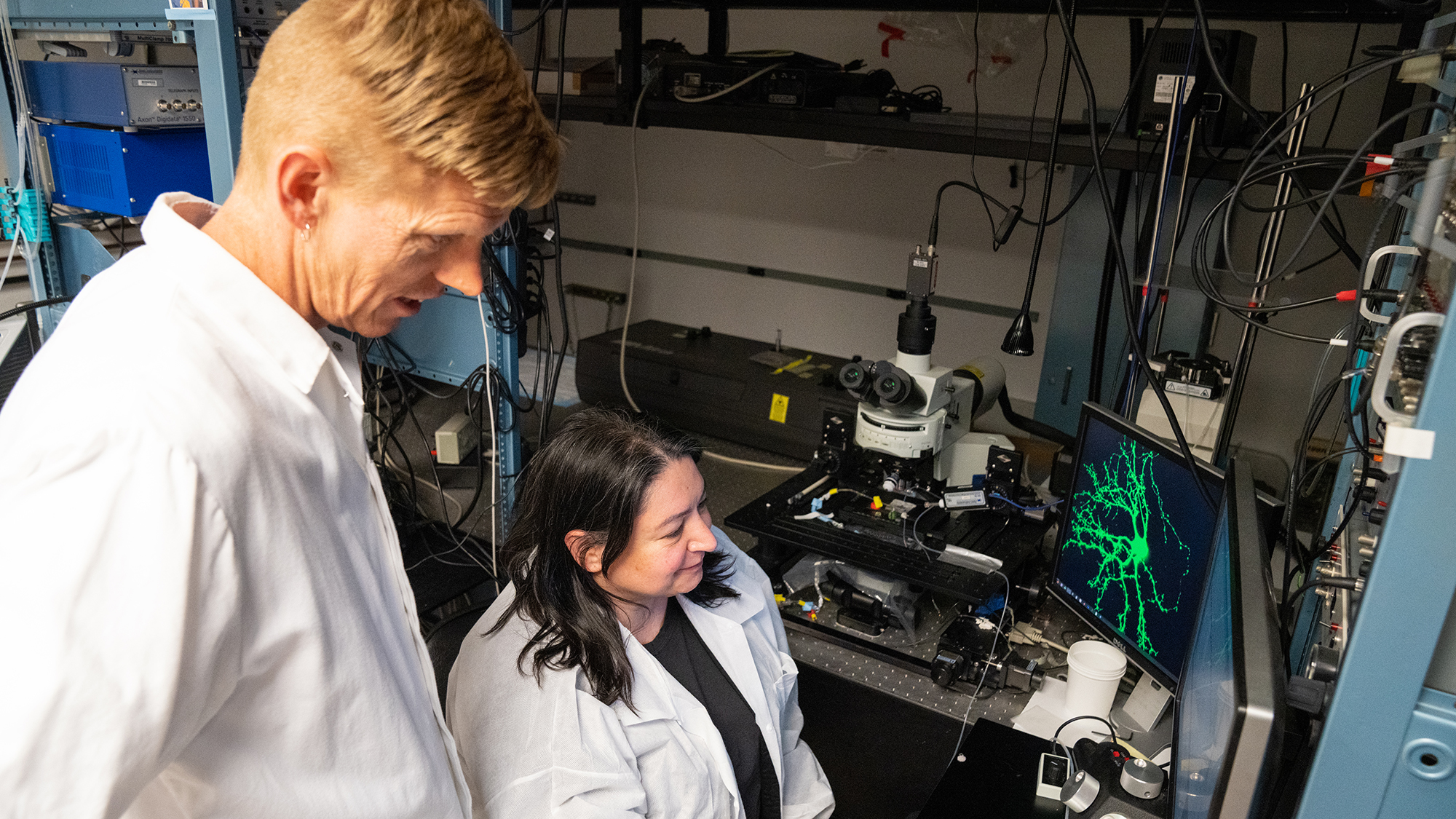
Surprising discoveries in autism
Researchers long believed that autism involved genes that regulate communications between neurons — especially the synapses, where neurotransmitters carry signals from one neuron to another. But starting in the early 2010s, genetic studies revealed that many autism risk genes actually play a very different role: they encode proteins that reside far from the synapses — in the cell ’s nucleus, where the DNA is located, and regulate the activity of hundreds of other genes.
“ This was a huge surprise , ” says Nord. Chd8 , a typical member of this group, regulates how tightly DNA is packaged and coiled with proteins. As such, it may regulate many genes that govern the formation and function of synapses.
At the same time, another major area of study is advancing the field of autism research. Scientists had assumed that autism was driven by changes in the cerebral cortex, which performs all sorts of tasks, such as recognizing words and faces, and “executive function” — controlling working memory, guiding our spotlight of attention, and making choices — such as whether to act on the impulse to withdraw one's hand when an unfamiliar dog approaches.
But in 2012, researchers reported that mice with mild abnormalities in the cerebellum developed behaviors that resembled autism in humans, such as reduced social interaction with other mice. “ The autism field was really rocked by this discovery , ” says Fioravante.
People had previously believed that the cerebellum mainly coordinates body movements such as walking, speaking, and typing. “ When you damage the cerebellum, it causes profound movement problems, and this probably made it difficult to see more subtle changes in behavior , ” says Fioravante.
But she points out that the cerebellum has significant connections with brain structures like the prefrontal cortex, which guides executive function, and limbic system, which regulates sociability, mood and emotions . People with cerebellar injuries often show autism-like changes in their emotions and social interactions.
Then in 2017, Nord and his colleagues reported a discovery that tied together these two intriguing threads : they found that the autism risk gene Chd8 helped guide the development of the cerebellum. Mice with one non-functioning copy of the gene had smaller cerebella.
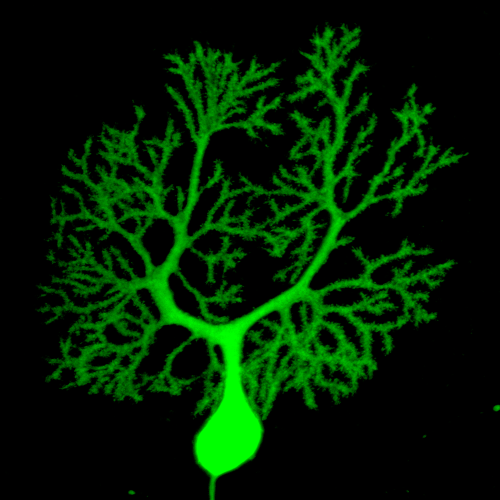
That discovery “ planted the idea of our project , ” says Fioravante. She and Nord sat in offices next door to one another. And while Nord studied Chd8 , she worked on the cerebellum. In 2021 they applied for, and received, an earlier NIMH grant, to study how Chd8 influences the development of the cerebellum in mice, before and shortly after birth. Fioravante also received a pilot grant from the Behavioral Health Center of Excellence at UC Davis that launched Chd8 cerebellar studies in adult mice.
Working on these earlier grants from 2021 to 2023, they found that Chd8 mutations in mice triggered changes in the cerebellum and in behavior that resemble what is seen in humans with autism. For example, mice with a mutant copy of the gene had impaired social cognition. While regular mice prefer to explore and interact with mice they have not met before, mice with mutant Chd8 had more restricted interests — preferring mice or objects that they already knew.
New targets for treatment
With the new grant, Fioravante and Nord will pick up where they left off. In adult mice with normally developed cerebella, they will use genetic tools to disrupt Chd8 . They will examine how loss of Chd8 alters gene expression and function in neurons of the cerebellum, and how the connections between it and other brain areas change. They will also study whether disruption of Chd8 causes autism-like behavioral changes, such as reduced social interaction with other mice, or reduced interest in novelty. Cesar Canales, an assistant professional researcher in NPB, who has expertise in cerebellar anatomy, will take part in these studies.
These experiments “will help shed light on the totality of what the cerebellum does , ” says Fioravante — getting beyond the traditional narrow view that it mainly coordinates movement. The team also hopes to uncover new strategies for treating autism.
Although autism involves early brain development, the condition usually isn ’t diagnosed until children are years older — when the abnormal brain connections are already established, potentially making treatment difficult.
In these upcoming studies, Nord and Fioravante hope to explore whether the treatment window for autism can be extended. “ If we see changes in behavior or neural circuits when Chd8 is disrupted in the developed cerebellum, then we know there is a treatment target , ” says Nord.
These studies could also shed light on schizophrenia and obsessive-compulsive disorder, which occur more frequently in people with mutations in this gene, says Nord: “ It ’s a molecular handle , an entry point into the pathophysiology of these other complex diseases . ”
Media Resources
- Douglas Fox is a freelance science writer based in the Bay Area.
- Fioravante Lab
- Germline Chd8 haploinsufficiency alters brain development in mouse ( Nature Neuroscience 2017)
- Cognitive-affective functions of the cerebellum ( The Journal of Neuroscience 2023)
Primary Category
Secondary categories.
- Alzheimer's & Dementia
- Asthma & Allergies
- Atopic Dermatitis
- Breast Cancer
- Cardiovascular Health
- Environment & Sustainability
- Exercise & Fitness
- Headache & Migraine
- Health Equity
- HIV & AIDS
- Human Biology
- Men's Health
- Mental Health
- Multiple Sclerosis (MS)
- Parkinson's Disease
- Psoriatic Arthritis
- Sexual Health
- Ulcerative Colitis
- Women's Health
- Nutrition & Fitness
- Vitamins & Supplements
- At-Home Testing
- Men’s Health
- Women’s Health
- Latest News
- Medical Myths
- Honest Nutrition
- Through My Eyes
- New Normal Health
- 2023 in medicine
- Why exercise is key to living a long and healthy life
- What do we know about the gut microbiome in IBD?
- My podcast changed me
- Can 'biological race' explain disparities in health?
- Why Parkinson's research is zooming in on the gut
- Health Hubs
- Find a Doctor
- BMI Calculators and Charts
- Blood Pressure Chart: Ranges and Guide
- Breast Cancer: Self-Examination Guide
- Sleep Calculator
- RA Myths vs Facts
- Type 2 Diabetes: Managing Blood Sugar
- Ankylosing Spondylitis Pain: Fact or Fiction
- Our Editorial Process
- Content Integrity
- Conscious Language
- Health Conditions
- Health Products
What is the latest research on autism?

Doctors have defined autism spectrum disorder (ASD) as a neurobiological developmental condition that can impact communication, sensory processing, and social interactions. Although recent research has advanced the understanding of autism, there is much more to learn about the factors that influence this neurotype.

As of March 26, 2021, the Centers for Disease Control and Prevention (CDC) report that among 8-year-old children, one in 54 are autistic. This number has increased from the one in 59 prevalence reported in previous estimates.
With autism rates on the increase, the scientific community has become all the more interested in uncovering the factors linked with autism.
Some scientists speculate that gene variants cause autism, while others believe environmental factors , such as exposure to toxins , contribute to this neurotype. Still others theorize imbalances in the intestinal microbiome may be at play.
The latest autism research includes investigations into factors associated with this neurotype , as well as genetic variants, gut biome imbalances, and neurological factors that may contribute to it.
In this Special Feature, Medical News Today examines the latest scientific discoveries and what researchers have learned about autism.
Exploring contributing factors
A multiyear study funded by the CDC is underway to learn more about factors potentially linked to autism.
The Study to Explore Early Development is a collaboration between six study sites in the United States. These sites are part of the Autism and Developmental Disabilities Research and Epidemiology network and focus on children aged 2–5 years.
One of the goals of the study is discovering what health conditions occur in autistic and neurotypical children and what factors are associated with the likelihood of developing ASD.
Another objective of the study is to differentiate the physical and behavioral characteristics of autistic children, children with other developmental conditions, and those without these conditions.
This ongoing research has already produced several published studies. The latest found an association between ASD and a mother’s exposure to ozone pollution during the third trimester of pregnancy.
Researchers also found that exposure to another type of air pollution called particulate matter during an infant’s first year also increased the likelihood of the infant later receiving a diagnosis of ASD.
This research appears in the journal Epidemiology .
Current research on genes
Other avenues of research on autism include investigations into gene variants that could play a role in the development of ASD.
A recent study analyzed the DNA of more than 35,584 people worldwide, including 11,986 autistic individuals. The scientists identified variants in 102 genes linked with an increased probability of developing ASD.
The researchers also discovered that 53 of the genes identified were mostly associated with autism and not other developmental conditions.
Expanding the research further, the team found that autistic people who carried the ASD-specific gene variants showed increased intellectual function compared with autistic individuals who did not have the variants.
The gene variants the scientists identified mainly reside in the cerebral cortex, which is responsible for complex behaviors.
These variants may play a role in how the brain neurons connect and also help turn other genes on or off — a possible factor that may contribute to autism.
Investigating neurological factors
Biological research has unearthed some interesting findings linking certain types of cell malfunctions to ASD.
Scientists at the Lieber Institute for Brain Development in Baltimore, MD, discovered a decrease in the integrity of myelin, a protective sheath surrounding nerve cells in the brain, in mice with a syndromic form of ASD.
The study, published in Nature Neuroscience , showed a gene variant-based malfunction in oligodendrocytes , which are cells that produce myelin.
This malfunction may lead to insufficient myelin production in the nerve cells and disrupt nerve communication in the brain, impairing brain development.
Using mouse models, researchers are now investigating treatments that could increase the myelination in the brain to see whether this improves ASD-associated behaviors that individuals may find challenging.
Autism and the gut microbiome
The gastrointestinal, or gut, microbiome is another area of interest to researchers looking for factors that contribute to autism.
Several studies have established a link between imbalances in the gut biome and ASD. There is also growing evidence that balancing the populations of gut microbes can help correct these disparities and improve some of the unwanted symptoms and behaviors linked to autism.
One 2017 study, published in the journal Microbiome , investigated whether microbiota transfer therapy (MTT) in autistic children improved gut microbiota diversity and symptoms associated with autism.
Investigators found that, after the MTT treatment, participants experienced more gut bacterial diversity.
Also observed in the participants treated with MTT was a decrease in gastrointestinal (GI) symptoms, as well as improved language, social interaction, and behavioral symptoms.
In a 2-year follow-up investigation , researchers found that the participants who received MTT treatment still experienced fewer GI issues and a continued improvement of autism-related symptoms.
Scientists have also recently discovered a possible connection between genes and the gut microbiome.
A study published earlier this month found that mice that lack CNTNAP2, a gene linked to autism, have an unusual population of microbes in their intestines. They also displayed some social behaviors similar to those seen in some autistic people.
When the mice were treated with Lactobacillus reuteri , a common bacterium missing from their microbiome, and a strain of gut bacteria commonly found in wild-type mice, their social behaviors improved.
New horizon of diagnostics and testing
Autism can be challenging to detect, especially in very young children. Research has shown early diagnosis and treatment interventions can lead to better long-term outcomes for autistic people.
Because of this, the scientific community is working toward finding innovative diagnostic methods that can help detect this neurotype much earlier.
Hearing tests may be one such diagnostic tool. Researchers from Harvard Medical School in Boston, MA, and the University of Miami analyzed data from auditory brainstem response (ABR) hearing tests routinely given to infants shortly after birth in the state of Florida.
The team then matched the data to the Florida Department of Education records of those children who later received a diagnosis of a developmental condition.
Results showed that infants who later received an ASD diagnosis had slower brain responses to sounds during their ABR tests conducted at birth.
This study appears in the journal Autism Research .
The investigators hope to conduct more studies to determine whether the ABR test could help recognize autism at an early age.
Further advancements in recognizing autism include new research into biomarkers.
When analyzing data from the Children’s Autism Metabolome Project (CAMP) , a team of researchers found metabotypes associated with autism in 357 children aged 18–48 months.
After optimizing these and previously discovered metabotypes into screening tests, the research team detected autism in 53% of the participants in the CAMP study.
Study author Elizabeth L. R. Donley of Stemina Biomarker Discovery in Madison, WI, told MNT :
“Our approach to understanding the biology of autism is going to revolutionize how we diagnose and treat autism. Autism is diagnosed through behavioral assessment, but there are underlying biological reasons for the disruptions in neurodevelopment that result in the behaviors of autism.”
Donley said the differences her team has identified in the metabolism of autistic children can provide insight into more specific treatment options where necessary.
“The first metabolic subtypes we published from our clinical study, the [CAMP], may be addressable with a supplement. The biology of other subtypes may be targets for drugs or new indications for existing drugs,” Donley explained.
She added, “[O]ur approach identifies where dysregulation is occurring in the biology of the child so that therapies that address this biology can be prioritized rather than just trying anything and everything with no precision.”
The research team has already validated the first three of five planned panels that can identify subtypes of metabolism associated with autism. They expect to validate the remaining panels this year and begin the first clinical study of a paired therapy.
With the prevalence of autism on the rise, scientists persevere to uncover what factors are associated with this neurotype.
Their hope is that, once they identify causative factors, researchers could then develop screening tests for earlier detection and more targeted treatments for symptoms and health conditions related to autism.
At the same time, nonprofit advocacy organizations run by autistic people, such as the Autistic Self Advocacy Network (ASAN ) , warn against regarding autism itself as something to be “treated” or “cured.”
The ASAN states that “[m]ost self-advocates agree that autism does not need to be cured. Instead of wasting time and money on something that is not possible and that autistic people do not want, we should focus on supporting autistic people to live good lives.”
“The most important thing,” the ASAN adds, “is that any therapy should help autistic people get what we want and need, not what other people think we need. Good therapies focus on helping us figure out our goals, and work with us to achieve them.”
- Neurology / Neuroscience
- Pediatrics / Children's Health
Share this article
Latest news
Related coverage.
'You don’t seem autistic' is something that I hear a lot. My name is Jonathan Rowland, and this is my experience of living with autism.
Contributor Kimberly Drake writes about her experience as an advocate for autism in her community, showing how real change can be built from the…
In the past, doctors referred to four different types of autism. However, doctors now diagnose autism, or autism spectrum disorder, in levels, and not…
Children with autism often experience developmental delays, but missing a developmental milestone does not always indicate autism. Learn more in this…
Sensory processing disorder and autism are separate conditions that can occur together. They can both cause issues in daily activities and…
Stanford-Only View
Stanford Autism Center
Autism research studies at stanford, study title/ age , description, spark (simons powering autism research) study.
If you or your child has a professional diagnosis of autism, Stanford University invites you to learn more about SPARK, a new online research study sponsored by the Simons Foundation Autism Research Initiative. The mission of SPARK is clear: speed up research and advance understanding of autism by creating the nation’s largest autism study. Joining SPARK is simple – register online and provide a DNA sample via a saliva collection kit in the comfort of your own home. Together, we can help spark a better future for all individuals and families affected by autism.
Register in person at Stanford University by contacting us at [email protected] or online at www.sparkforautism.org/stanford .
Targeting the neurobiology of restricted and repetitive behaviors in children with autism using N-acetylcysteine: Randomized Controlled Trial
3-12 Years
We are recruiting children with autism spectrum disorder to participate in a research study at Stanford University. Our goal is to examineth effects of N-acetyl cysteine, an over-the-counter dietary supplement, on the brain circuits that underlie some restricted and repetitive behaviors.
To be elligible for this trial, your child must:
- be aged between 3 and 12 years old
- exhibit restricted and repetitive behaviors
- be willing to drink N-acetyl cysteine dissolved in water
- be willing to undergo brain scanning with magnetic resonance imaging (MRI)
- be willing to undergo brain scanning with electroencephalography (EEG)
The study will take place at Stanford University over 12-to-16-week period. Our safety protocols have been updated for COVID-19 and many research activities will be completed remotely using Zoom and virtual surveys. Your child must be willing to:
- complete cognitive and behvaiorial assessments (such as IQ tetsing)
- be able to either sleep (young children) or lie still in the scanner during an MRI
- tolerate wearing an EEG cap
- drink N-acetyl cysteine dissolved in water for a total of 12-week period
For Participant inquiries contact: [email protected]
Autism Center of Excellence Sleep Study
8-17 Years
Dear Parents,
We are excited to tell you about a new research study for children. We are looking to partner with parents who have children that are between the ages of 4 and 17 years old, with and without an Autism Spectrum Disorder (ASD) diagnosis.
What is involved?
- In-person cognitive and behavioral assessments
- Day-time Electroencephalogram (EEG)
- In-home, 2 night sleep monitoring session
- Collection of saliva to measure cortisol and melatonin levels
- Wearing a watch device that tracks sleep and daily activity
What will I receive if I participate?
- Research sleep report and behavioral testing summary upon request
- $50 for each in-person visit to Stanford and $100 for the 2 night in-home sleep assessment
Treatment extension study:
- If your child has ASD, sleep difficulties, and ages 8-17, they may also qualify for sleep medication trials
Interested in participating or want to learn more? Click Here!
If you would like to reach out to our team directly with any questions, please contact our team below!
Email: [email protected]
650-498-7215
Neuroimaging Predictors of Improvement to Pivotal Response Treatment (PRT) in Young Children With Autism
Stanford University researchers are recruiting children with autism to identify brain imaging predictors of benefits from Pivotal Response Treatment (PRT) targeting language abilities.
In order to participate in this research study, your child must:
- Be between the ages of 2 and 4 years
- Be able to complete an MRI of the brain during natural sleep
- Participate in a 16-week parent training program
- Meet inclusion based on testing.
Vasopressin Treatment Trial for Children with Autism
6 - 17 years
The purpose of this clinical trial is to investigate the effectiveness of vasopressin nasal spray for treating symptoms associated with autism. Vasopressin is a hormone that is produced naturally within the body and has been implicated in regulating social behaviors. It has been proposed that administration of the hormone may also help improve social functioning in individuals with autism.
Link to study at clinicaltrials.gov
Pregnenolone Randomized Controlled Trial
14 - 21 years
Neurosteroid Pregnenolone Treatment for Irritability in Adolescents with Autism
Medication treatments for core symptoms of autism spectrum disorder (ASD) continue to be unmet medical needs. The only medications approved by the U.S. Food and Drug Administration (FDA) for the treatment of individuals with ASD are effective in treating irritability and associated aggressive behaviors, but these medications can also cause severe long-term side effects such as diabetes and involuntary motor movements. Therefore, effective medications with more tolerable side effect profiles are highly desirable. This profile is consistent with pregnenolone (PREG). PREG belongs to a new class of hormones known as neurosteroids, which have been shown to be effective in treating various psychiatric conditions including bipolar depression and schizophrenia. As compared to currently FDA-approved medications, our preliminary data suggested that PREG may represent a potentially effective and well-tolerated agent for treating irritability in individuals with ASD. In addition, our experience suggests that PREG might be helpful in improving selected core symptoms such as social deficits and sensory abnormalities of ASD. This study provides the opportunity to further explore the usefulness of PREG in the treatment of irritability and some core symptoms of ASD. We are performing a 12-week randomized double-blind controlled pilot trial to examine the effectiveness of orally administered PREG in reducing irritability and associated behaviors in adolescents with ASD. In this study, we also aim to examine the usefulness of biomarkers (blood levels of neurosteroids, eyetracking and brain wave recording) in predicting treatment response and assessing biologic changes with PREG treatment.
Study Flyer
Link to study in Stanford's Clinical Trials Directory
Helpful Links
- Development-Behavioral Pediatrics at Stanford Children's Health
- Divison of Child & Adolescent Psychiatry and Child Development
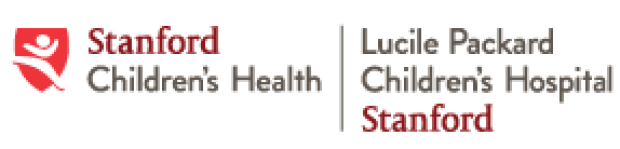
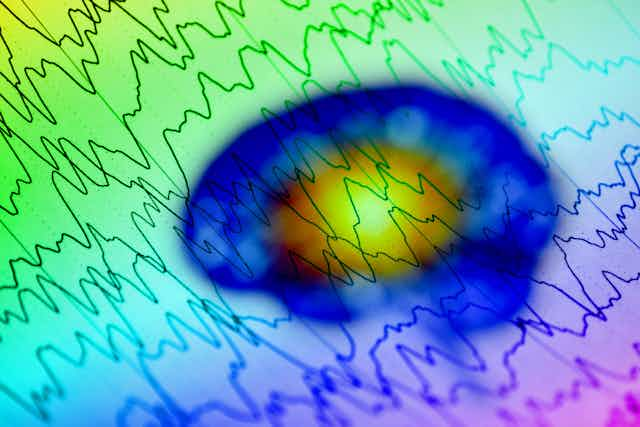
‘Noisy’ autistic brains seem better at certain tasks. Here’s why neuroaffirmative research matters
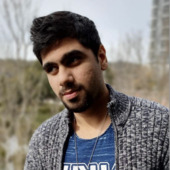
PhD candidiate, University of Canberra
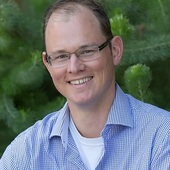
Associate professor, University of Canberra
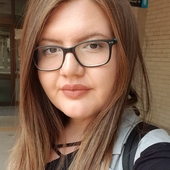
Honours Graduate Student, University of Canberra
Disclosure statement
Jeroen van Boxtel receives funding from Australian Government through an Australian Research Council Discovery Project (project number DP220100406), the ACT government.
Jovana Acevska and Pratik Raul do not work for, consult, own shares in or receive funding from any company or organisation that would benefit from this article, and have disclosed no relevant affiliations beyond their academic appointment.
University of Canberra provides funding as a member of The Conversation AU.
View all partners
Autism is a neurodevelopmental difference associated with specific experiences and characteristics.
For decades, autism research has focused on behavioural, cognitive, social and communication difficulties. These studies highlighted how autistic people face issues with everyday tasks that allistic (meaning non-autistic) people do not. Some difficulties may include recognising emotions or social cues.
But some research, including our own study, has explored specific advantages in autism. Studies have shown that in some cognitive tasks, autistic people perform better than allistic people. Autistic people may have greater success in identifying a simple shape embedded within a more complex design , arranging blocks of different shapes and colours , or spotting an object within a cluttered visual environment (similar to Where’s Wally?). Such enhanced performance has been recorded in babies as young as nine months who show emerging signs of autism.
How and why do autistic individuals do so well on these tasks? The answer may be surprising: more “neural noise”.
Read more: From deficits to a spectrum, thinking around autism has changed. Now there are calls for a 'profound autism' diagnosis
What is neural noise?
Generally, when you think of noise, you probably think of auditory noise, the ups and downs in the amplitude of sound frequencies we hear.
A similar thing happens in the brain with random fluctuations in neural activity. This is called neural noise.
This noise is always present, and comes on top of any brain activity caused by things we see, hear, smell and touch. This means that in the brain, an identical stimulus that is presented multiple times won’t cause exactly the same activity. Sometimes the brain is more active, sometimes less. In fact, even the response to a single stimulus or event will fluctuate continuously.
Neural noise in autism
There are many sources of neural noise in the brain. These include how the neurons become excited and calm again, changes in attention and arousal levels, and biochemical processes at the cellular level, among others. An allistic brain has mechanisms to manage and use this noise . For instance, cells in the hippocampus (the brain’s memory system) can make use of neural noise to enhance memory encoding and recall.
Evidence for high neural noise in autism can be seen in electroencephalography (EEG) recordings , where increased levels of neural fluctuations were observed in autistic children. This means their neural activity is less predictable, showing a wider range of activity (higher ups and downs) in response to the same stimulus.
In simple terms, if we imagine the EEG responses like a sound wave, we would expect to see small ups and downs (amplitude) in allistic brains each time they encounter a stimulus. But autistic brains seem to show bigger ups and downs, demonstrating greater amplitude of neural noise.
Many studies have linked this noisy autistic brain with cognitive, social and behavioural difficulties .
Read more: Most adults with autism can recognise facial emotions, almost as well as those without the condition
But could noise be a bonus?
The diagnosis of autism has a long clinical history . A shift from the medical to a more social model has also seen advocacy for it to be reframed as a difference, rather than a disorder or deficit. This change has also entered autism research. Neuroaffirming research can examine the uniqueness and strengths of neurodivergence.
Psychology and perception researcher David Simmons and colleagues at the University of Glasgow were the first to suggest that while high neural noise is generally a disadvantage in autism, it can sometimes provide benefits due to a phenomenon called stochastic resonance . This is where optimal amounts of noise can enhance performance . In line with this theory, high neural noise in the autistic brain might enhance performance for some cognitive tasks.
Our 2023 research explores this idea . We recruited participants from the general population and investigated their performance on letter-detection tasks. At the same time, we measured their level of autistic traits.
We performed two letter-detection experiments (one in a lab and one online) where participants had to identify a letter when displayed among background visual static of various intensities.
By using the static, we added additional visual noise to the neural noise already present in our participants’ brains. We hypothesised the visual noise would push participants with low internal brain noise (or low autistic traits) to perform better (as suggested by previous research on stochastic resonance). The more interesting prediction was that noise would not help individuals who already had a lot of brain noise (that is, those with high autistic traits), because their own neural noise already ensured optimal performance.
Indeed, one of our experiments showed people with high neural noise (high autistic traits) did not benefit from additional noise. Moreover, they showed superior performance (greater accuracy) relative to people with low neural noise when the added visual static was low. This suggests their own neural noise already caused a natural stochastic resonance effect, resulting in better performance.
It is important to note we did not include clinically diagnosed autistic participants, but overall, we showed the theory of enhanced performance due to stochastic resonance in autism has merits.
Read more: Autism is still underdiagnosed in girls and women. That can compound the challenges they face
Why this is important?
Autistic people face ignorance, prejudice and discrimination that can harm wellbeing . Poor mental and physical health, reduced social connections and increased “camouflaging” of autistic traits are some of the negative impacts that autistic people face.
So, research underlining and investigating the strengths inherent in autism can help reduce stigma, allow autistic people to be themselves and acknowledge autistic people do not require “fixing”.
The autistic brain is different. It comes with limitations, but it also has its strengths.
- Autism research
- Disability coverage

Events Officer

Lecturer (Hindi-Urdu)

Director, Defence and Security

Opportunities with the new CIEHF

School of Social Sciences – Public Policy and International Relations opportunities

An official website of the United States government
The .gov means it’s official. Federal government websites often end in .gov or .mil. Before sharing sensitive information, make sure you’re on a federal government site.
The site is secure. The https:// ensures that you are connecting to the official website and that any information you provide is encrypted and transmitted securely.
- Publications
- Account settings
Preview improvements coming to the PMC website in October 2024. Learn More or Try it out now .
- Advanced Search
- Journal List
- Cambridge Open

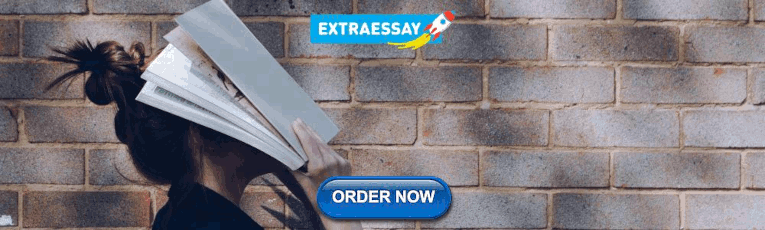
Genetic contributions to autism spectrum disorder
1 Nic Waals Institute, Lovisenberg Diaconal Hospital, Oslo, Norway
2 Department of Mental Disorders, Norwegian Institute of Public Health, Oslo, Norway
3 Department of Psychology, PROMENTA Research Center, University of Oslo, Oslo, Norway
M. Niarchou
4 Vanderbilt Genetics Institute, Vanderbilt University Medical Center, TN, USA
A. Starnawska
5 The Lundbeck Foundation Initiative for Integrative Psychiatric Research, iPSYCH, Denmark
6 Department of Biomedicine, Aarhus University, Denmark
7 Center for Genomics for Personalized Medicine, CGPM, and Center for Integrative Sequencing, iSEQ, Aarhus, Denmark
8 College of Medicine, Mohammed Bin Rashid University of Medicine and Health Sciences, Dubai, UAE
C. van der Merwe
9 Stanley Center for Psychiatric Research, The Broad Institute of MIT and Harvard, MA, USA
10 Department of Psychiatry, Autism Research Centre, University of Cambridge, UK
Autism spectrum disorder (autism) is a heterogeneous group of neurodevelopmental conditions characterized by early childhood-onset impairments in communication and social interaction alongside restricted and repetitive behaviors and interests. This review summarizes recent developments in human genetics research in autism, complemented by epigenetic and transcriptomic findings. The clinical heterogeneity of autism is mirrored by a complex genetic architecture involving several types of common and rare variants, ranging from point mutations to large copy number variants, and either inherited or spontaneous ( de novo ). More than 100 risk genes have been implicated by rare, often de novo , potentially damaging mutations in highly constrained genes. These account for substantial individual risk but a small proportion of the population risk. In contrast, most of the genetic risk is attributable to common inherited variants acting en masse , each individually with small effects. Studies have identified a handful of robustly associated common variants. Different risk genes converge on the same mechanisms, such as gene regulation and synaptic connectivity. These mechanisms are also implicated by genes that are epigenetically and transcriptionally dysregulated in autism. Major challenges to understanding the biological mechanisms include substantial phenotypic heterogeneity, large locus heterogeneity, variable penetrance, and widespread pleiotropy. Considerable increases in sample sizes are needed to better understand the hundreds or thousands of common and rare genetic variants involved. Future research should integrate common and rare variant research, multi-omics data including genomics, epigenomics, and transcriptomics, and refined phenotype assessment with multidimensional and longitudinal measures.
Definition of autism
Kanner defined autism in 1943 with detailed case descriptions of children showing social aloofness, communication impairments, and stereotyped behaviors and interests, often accompanied by intellectual disability (ID) (Kanner, 1943 ). A year later, Asperger independently published an article on children presenting marked difficulties in social communication and unusually circumscribed and intense interests, despite advanced intellectual and language skills (Asperger, 1944 ). Three decades later, Wing and Gould united Asperger and Kanner's descriptions and conceptualized a spectrum of autistic conditions (Wing and Gould, 1978 , 1979 ).
The onset of autism is during the first years of life, although symptoms may not be fully apparent or recognized until later (American Psychiatric Association, 2013 ). Autism is a heterogeneous and complex group of conditions with considerable variation in core symptoms, language level, intellectual functioning, and co-occurring psychiatric and medical difficulties. Subtype diagnoses such as childhood autism and Asperger's syndrome were previously used to specify more homogeneous presentations, but were unstable over time within individuals and used unreliably by clinicians (Lord et al., 2020 ). Current editions of the major diagnostic manuals have replaced the subtypes with an overarching autism spectrum disorder diagnosis and instead require specification of key sources of heterogeneity; language level, intellectual functioning, and co-occurring conditions (APA, 2013 ; World Health Organization, 2018 ).
Epidemiology
Prevalence estimates of autism have steadily increased from less than 0.4% in the 1970s to current estimates of 1–2% (Fombonne, 2018 ; Lyall et al., 2017 ). The increase is largely explained by broadening diagnostic criteria to individuals without ID and with milder impairments, and increased awareness and recognition of autistic traits (Lord et al., 2020 ; Taylor et al., 2020 ). There are marked sex and gender differences in autism (Halladay et al., 2015 ; Warrier et al., 2020 ). The male-to-female ratio is approximately 4:1 in clinical and health registry cohorts but closer to 3:1 in general population studies with active case-finding (Loomes, Hull, & Mandy, 2017 ) and 1–2:1 in individuals with moderate-to-severe ID (Fombonne, 1999 ; Yeargin-Allsopp et al., 2003 ). The mechanisms underlying the sex difference are mostly unknown, and hypotheses include a female protective effect (aspects of the female sex conferring resilience to risk factors for autism), prenatal steroid hormone exposure, and social factors such as underdiagnosis and misdiagnosis in women (Ferri, Abel, & Brodkin, 2018 ; Halladay et al., 2015 ).
Co-occurring conditions are the rule rather than the exception, estimated to affect at least 70% of people with autism from childhood (Lai et al., 2019 ; Simonoff et al., 2008 ). Common co-occurring conditions include attention-deficit hyperactivity disorder (ADHD), anxiety, depression, epilepsy, sleep problems, gastrointestinal and immune conditions (Davignon, Qian, Massolo, & Croen, 2018 ; Warrier et al., 2020 ). There is an elevated risk of premature mortality from various causes, including medical comorbidities, accidental injury, and suicide (Hirvikoski et al., 2016 ).
Autism is also associated with positive traits such as attention to detail and pattern recognition (Baron-Cohen & Lombardo, 2017 ; Bury, Hedley, Uljarević, & Gal, 2020 ). Further, there is wide variability in course and adulthood outcomes with regard to independence, social relationships, employment, quality of life, and happiness (Howlin & Magiati, 2017 ; Mason et al., 2020 ; Pickles, McCauley, Pepa, Huerta, & Lord, 2020 ). Rigorous longitudinal studies and causally informative designs are needed to determine the factors affecting developmental trajectories and outcomes.
Environmental factors
Twin studies suggest that 9–36% of the variance in autism predisposition might be explained by environmental factors (Tick, Bolton, Happé, Rutter, & Rijsdijk, 2016 ). There is observational evidence for association with pre- and perinatal factors such as parental age, asphyxia-related birth complications, preterm birth, maternal obesity, gestational diabetes, short inter-pregnancy interval, and valproate use (Lyall et al., 2017 ; Modabbernia, Velthorst, & Reichenberg, 2017 ). Mixed results are reported for pregnancy-related nutritional factors and exposure to heavy metals, air pollution, and pesticides, while there is strong evidence that autism risk is unrelated to vaccination, maternal smoking, or thimerosal exposure (Modabbernia et al., 2017 ). It is challenging to infer causality from observed associations, given that confounding by lifestyle, socioeconomic, or genetic factors contributes to non-causal associations between exposures and autism. Many putative exposures are associated with parental genotype (e.g. obesity, age at birth) (Gratten et al., 2016 ; Taylor et al., 2019a , Yengo et al., 2018 ), and some are associated both with maternal and fetal genotypes (e.g. preterm birth) (Zhang et al., 2017 ). Studies triangulating genetically informative designs are needed to disentangle these relationships (Davies et al., 2019 ; Leppert et al., 2019 ; Thapar & Rutter, 2019 ).
Twin and pedigree studies
In 1944, Kanner noted that parents shared common traits with their autistic children, introducing the ‘broader autism phenotype’ (i.e. sub-threshold autistic traits) and recognizing the importance of genetics (Harris, 2018 ; Kanner, 1944 ). Thirty years later, twin studies revolutionized the field of autism research (Ronald & Hoekstra, 2011 ).
Twin studies were the first to demonstrate the heritability of autism. In 1977, the first twin-heritability estimate was published, based on a study of 10 dizygotic (DZ) and 11 monozygotic (MZ) pairs (Folstein & Rutter, 1977 ). Four out of the 11 MZ pairs (36%) but none of the DZ pairs were concordant for autism. Subsequently, over 30 twin studies have been published, further supporting the high heritability of autism (Ronald & Hoekstra, 2011 ). A meta-analysis of seven primary twin studies reported that the heritability estimates ranged from 64% to 93% (Tick et al., 2016 ). The correlations for MZ twins were at 0.98 [95% confidence interval (CI) 0.96–0.99], while the correlations for DZ twins were at 0.53 (95% CI 0.44–0.60) when the autism prevalence rate was assumed to be 5% (based on the broader autism phenotype) and increased to 0.67 (95% CI 0.61–0.72) when the prevalence was 1% (based on the stricter definition) (Tick et al., 2016 ). Additionally, family studies have found that the relative risk of a child having autism relates to the amount of shared genome with affected relatives ( Fig. 1 ) (Bai et al., 2019 ; Constantino et al., 2013 ; Georgiades et al., 2013 ; Grønborg, Schendel, & Parner, 2013 ; Risch et al., 2014 ; Sandin et al., 2014 ).

Relative risk of autism by degree of relatedness with a person with autism. Relative risk for full and half siblings, and full cousins was provided in Hansen et al. ( 2019 ). Relative risk for half first cousins was estimated based on Xie et al. ( 2019 ). GS, genome shared.
Early twin and pedigree studies demonstrated that the biological relatives of individuals with autism who did not meet the criteria for an autism diagnosis themselves commonly showed elevated autistic traits such as communication and social interaction difficulties (Le Couteur et al., 1996 ), indicating that the heritability is not restricted to the traditional diagnostic boundaries of autism. Twin studies also indicate that although social communication and repetitive behavior trait dimensions each show strong heritability, there is a limited genetic correlation between them (e.g. for a review, see Ronald & Hoekstra, 2011 ). Further, twin studies have found substantial genetic overlap between autistic traits and symptoms of other psychiatric conditions, including language delay (e.g. Dworzynski et al., 2008 ), ID (e.g. Nishiyama et al., 2009 ), ADHD (e.g. Ronald, Edelson, Asherson, & Saudino, 2010 ), and anxiety (e.g. Lundström et al., 2011 ) (for a review, see Ronald & Hoekstra, 2014 ). Moreover, twin and family studies indicate that the sibling recurrence rate of autism is lower in female than male siblings (Palmer et al., 2017 ; Werling & Geschwind, 2015 ), suggesting the female protective effect hypothesis as a potential explanation for the male preponderance in the diagnosis of autism. The hypothesis was supported by results showing that the siblings of autistic females had a higher likelihood of high autistic trait scores and autism than the siblings of autistic males (Ferri et al., 2018 ; Palmer et al., 2017 ; Robinson, Lichtenstein, Anckarsäter, Happé, & Ronald, 2013 ), consistent with females having a higher liability threshold.
Genetic variants differ in the frequency at which they occur in the population (e.g. rare v. common), the type (i.e. SNPs/CNVs/translocations and inversions/indels), and whether they are inherited or de novo . Here, we summarize the findings on genetic risk for autism from linkage and candidate gene studies, common and rare genetic variation studies, epigenomics, and transcriptomics. A glossary of important terms is in Box 1 .
Candidate gene association study: A study that examines the association between a phenotype and a genetic variant chosen a priori based on knowledge of the gene's biology or functional impact.
Complex trait: A trait that does not follow Mendelian inheritance patterns, but is likely the result of multiple factors including a complex mixture of variation within multiple genes.
Copy number variant (CNV): Deletion or duplication of large genomic regions.
de novo mutation: A mutation that is present in the offspring but is either absent in parents or is present only in parental germ cells.
DNA methylation (DNAm): Epigenetic modification of DNA characterized by the addition of a methyl group (-CH 3 ) to the 5 th position of the pyrimidine ring of cytosine base resulting in 5-methylcytosine (5mC).
Epigenetics: The science of heritable changes in gene regulation and expression that do not involve changes to the underlying DNA sequence.
Epigenome-Wide Association Study (EWAS): A study that investigates associations between DNA methylation levels quantified at tens/hundreds of thousands of sites across the human genome, and the trait of interest.
Genome-Wide Association Study (GWAS): A study scanning genome-wide genetic variants for associations with a given trait.
Genetic correlation: An estimate of the proportion of variance shared between two traits due to shared genetics.
Heritability: An estimate of the proportion of variation in a given trait that is due to differences in genetic variation between individuals in a given population.
Heritability on the liability scale : A heritability estimate adjusted for the population prevalence of a given binary trait, typically disorders.
Genetic linkage studies: A statistical method of mapping genes of heritable traits to their chromosomal locations by using chromosomal co-segregation with the phenotype.
Mendelian inheritance: When the inheritance of traits is passed down from parents to children and is controlled by a single gene for which one allele is dominant and the other recessive.
Methylation Quantitative Trait Locus (mQTL): A SNP at which genotype is correlated with the variation of DNA methylation levels at a nearby ( cis- mQTL) or distal ( trans- mQTL) site.
Phenotype: The observable characteristics of an individual.
Polygenic risk score (PRS): An estimate of an individual's genetic liability for a condition calculated based on the cumulative effect of many common genetic variants.
Single nucleotide polymorphism (SNP): A single base pair change that is common (>1%) in the population.
Single nucleotide variant (SNV): A variation in a single nucleotide without any limitation of frequency.
SNP heritability: The proportion of variance in a given phenotype in a population that is attributable to the additive effects of all SNPs tested. Typically, SNPs included have a minor allele frequency >1%.
Linkage and candidate gene studies
Initial linkage studies were conducted to identify chromosomal regions commonly inherited in affected individuals. Susceptibility loci implicated a range of regions, but only two have been replicated (Ramaswami & Geschwind, 2018 ): at chromosome 20p13 (Weiss, Arking, Daly, & Chakravarti, 2009 ) and chromosome 7q35 (Alarcón, Cantor, Liu, Gilliam, & Geschwind, 2002 ). Lack of replication and inconsistent findings were largely due to low statistical power (Kim & Leventhal, 2015 ). Candidate gene association studies identified over 100 positional and/or functional candidate genes for associations with autism (Bacchelli & Maestrini, 2006 ). However, there was no consistent replication for any of these findings (Warrier, Chee, Smith, Chakrabarti, & Baron-Cohen, 2015 ), likely due to limitations in study design (e.g. low statistical power, population diversity, incomplete coverage of variation within the candidate genes, and false positives arising from publication bias) (Ioannidis, 2005 ; Ioannidis, Ntzani, Trikalinos, & Contopoulos-Ioannidis, 2001 ). The advancement of genome-wide association studies (GWAS) and next-generation sequencing techniques has significantly enhanced gene and variant discovery.
Common genetic variation
The SNP-heritability (proportion of variance attributed to the additive effects of common genetic variants) of autism ranges from 65% in multiplex families (Klei et al., 2012 ) to 12% in the latest Psychiatric Genomics Consortium GWAS ( Fig. 2 a ) (Autism Spectrum Disorders Working Group of The Psychiatric Genomics Consortium, 2017 ; Grove et al., 2019 ). Variation is largely attributable to sample heterogeneity and differences in methods used to estimate SNP-heritability.

Variance explained by different classes of genetic variants in autism. ( a ) Donut chart of the variance explained by different classes of variants. The narrow-sense heritability (82.7%, Nordic average, shades of green) has been estimated using familial recurrence data from Bai et al. ( 2019 ). The total common inherited heritability (12%) has been estimated using LDSC-based SNP-heritability (additive) from Grove et al. ( 2019 ) and the total rare inherited heritability (3%) has been obtained from Gaugler et al. ( 2014 ). The currently unexplained additive heritability is thus 67.7% (total narrow-sense heritability minus common and rare inherited heritabilities combined). This leaves a total of 17.3% of the variance to shared and unique environmental estimates (Bai et al., 2019 ). The term environmental refers to non-additive and non-inherited factors that contribute to variation in autism liability. Of this, de novo missense and protein-truncating variants (Satterstrom et al., 2020 ) and variation in non-genic regions (An et al., 2018 ) together explain 2.5% of the variance. Whilst de novo variation can be inherited in some cases (germline mutation in the parent) and thus shared between siblings, it is unlikely that this will be shared by other related individuals, and thus unlikely to be included in the narrow-sense heritability in Bai et al. ( 2019 ). This is likely to be a lower-bound of the estimate as we have not included the variance explained by de novo structural variants and tandem repeats. Additionally, non-additive variation accounts for ~4% of the total variance (Autism Sequencing Consortium et al., 2019 ). Thus, ~11% of the total variance is currently unaccounted for, though this is likely to be an upper bound. ( b ) The variance explained is likely to change in phenotypic subgroups. For instance, the risk ratio for de novo protein-truncating variants in highly constrained genes (pLI > 0.9) is higher in autistic individuals with ID compared to those without ID (point estimates and 95% confidence intervals provided; Kosmicki et al., 2017 ). ( c ) Similarly, the proportion of the additive variance explained by common genetic variants is higher in autistic individuals without ID compared to autistic individuals with ID (Grove et al., 2019 ). Point estimates and 95% confidence intervals provided.
Early GWASs of autism were underpowered, partly due to overestimating potential effect sizes. Grove et al. ( 2019 ) conducted a large GWAS of autism combining data from over 18 000 autistic individuals and 27 000 non-autistic controls and an additional replication sample. They identified five independent GWAS loci ( Fig. 3 ). Another recent study (Matoba et al., 2020 ) identified a further novel locus by meta-analyzing the results from Grove et al. ( 2019 ) with over 6000 case-pseudocontrol pairs from the SPARK cohort by employing a massively parallel reporter assay to identify a potential causal variant (rs7001340) at this locus which regulates DDH2 in the fetal brain. The sample sizes are still relatively small compared to other psychiatric conditions (Schizophrenia Working Group of the Psychiatric Genomics Consortium, 2020 ; Howard et al., 2019 ), though ongoing work aims to double the sample size and identify additional loci.

Karyogram showing the 102 genes implicated by rare variant findings at a false discovery rate of 0.1 or less (Satterstrom et al., 2020 ) and the five index SNPs identified in GWAS (Grove et al., 2019 ) of autism.
Using genetic correlations and polygenic score analyses, studies have identified modest shared genetics between autism and different definitions of autistic traits in the general population (Askeland et al., 2020 ; Bralten et al., 2018 ; Robinson et al., 2016 ; Taylor et al., 2019 b ). There is some evidence for developmental effects, with greater shared genetics in childhood compared to adolescence (St Pourcain et al., 2018 ). These methods have also identified modest polygenic associations between autism and other neurodevelopmental and mental conditions such as schizophrenia, ADHD, and major depressive disorder, related traits such as age of walking, language delays, neuroticism, tiredness, and self-harm, as well as risk of exposure to childhood maltreatment and other stressful life events (Brainstorm Consortium et al., 2018 ; Bulik-Sullivan et al., 2015 ; Grove et al., 2019 ; Hannigan et al., 2020 ; Lee et al., 2019 , b ; Leppert et al., 2019 ; Cross-Disorder Group of the Psychiatric Genomics Consortium, 2013 ; Warrier & Baron-Cohen, 2019 ). Notably, autism is positively genetically correlated with measures of intelligence and educational attainment (EA) (Bulik-Sullivan et al., 2015 ; Grove et al., 2019 ), an observation supported by polygenic score association (Clarke et al., 2016 ). Polygenic Transmission Disequilibrium Tests have identified an over-transmission of polygenic scores for EA, schizophrenia, and self-harm from parents to autistic children, but an absence of such over-transmission to non-autistic siblings (Warrier & Baron-Cohen, 2019 ; Weiner et al., 2017 ), suggesting that these genetic correlations are not explained by ascertainment biases or population stratification. However, a genetic correlation does not necessarily imply a causal relationship between the two phenotypes and may simply index biological pleiotropy. Causal inference methods such as Mendelian randomization can be used to disentangle such relationships (Davies et al., 2019 ; Pingault et al., 2018 ).
The relatively low SNP-heritability in autism compared to other psychiatric conditions may partly be due to phenotypic heterogeneity. In an attempt to reduce phenotypic heterogeneity, Chaste et al. ( 2015 ) identified 10 phenotypic combinations to subgroup autistic individuals. Family-based association analyses did not identify significant loci, and SNP-heritability for the subgroups was negligent. It is unclear if reducing phenotypic heterogeneity increases genetic homogeneity, and investigating this in larger samples is warranted. Another study identified no robust evidence of genetic correlation between social and non-social (restricted and repetitive behavior patterns) autistic traits (Warrier et al., 2019 ). A few studies have investigated the common variant genetic architecture of social and non-social autistic traits in individuals with autism (Alarcón et al., 2002 ; Cannon et al., 2010 ; Cantor et al., 2018 ; Lowe, Werling, Constantino, Cantor, & Geschwind, 2015 ; Tao et al., 2016 ; Yousaf et al., 2020 ) and in the general population (St Pourcain et al., 2014 ; Warrier et al., 2018 , 2019 ), but replication of the identified loci is needed.
Diagnostic classification is another source of heterogeneity: SNP-heritability of Asperger's syndrome (ICD-10 diagnosis) was twice (0.097 ± 0.001) that of childhood autism and unspecified pervasive developmental disorders (Grove et al., 2019 ) [due to overlap in subtype diagnoses, a hierarchy was used: childhood autism>atypical autism>Asperger's syndrome>unspecified subtypes (Grove et al., 2019 )]. Supporting this, polygenic scores for intelligence and EA had larger loadings in the Asperger's syndrome and childhood autism subgroups compared to other subgroups (Grove et al., 2019 ). Additionally, the SNP-heritability of autism (all subtypes) without co-occurring ID diagnosis (0.09 ± 0.005) was three times that of autism with ID (Grove et al., 2019 ) ( Fig. 2 c ).
Rare genetic variation
Rare genetic variants confer significant risk in the complex etiology of autism. They are typically non-Mendelian, with substantial effect sizes and low population attributable risk. It is estimated that ~10% of autistic individuals have been diagnosed with an identifiable rare genetic syndrome characterized by dysmorphia, metabolic, and/or neurologic features (Carter & Scherer, 2013 ; Tammimies et al., 2015 ). Associated syndromes include the 15q11-q13 duplication of the Prader-Willi/Angelman syndrome, fragile X syndrome, 16p11.2 deletion syndrome, and 22q11 deletion syndrome (Sztainberg & Zoghbi, 2016 ). Prevalence estimates for autism vary widely between genetic syndromes; for example, 11% in 22q11.2 deletion syndrome and 54% in Cohen's syndrome (Richards, Jones, Groves, Moss, & Oliver, 2015 ). Of note, estimating the prevalence of autism in the context of genetic syndromes is complex (Havdahl et al., 2016 ; Richards et al., 2015 ).
The rate of gene discovery in autism is a linear function of increasing sample size (De Rubeis et al., 2014 ). Early studies implicated nine genes in the first 1000 autism cases (Neale et al., 2012 ; Sanders et al., 2012 ), increasing to 27 and 33 associated genes from separate analyses of Simons Simplex Collection and Autism Sequencing Consortium (ASC) samples (De Rubeis et al., 2014 ; Iossifov et al., 2014 ). Integrating these samples using the TADA framework implicated a total of 65 autism genes (Sanders et al., 2015 ).
The MSSNG initiative analyzed whole genomes from 5205 individuals ( N cases = 2636), and identified 61 autism-risk genes, of which 18 were new candidates (Yuen et al., 2017 ). More recently, the largest whole-exome sequencing analysis to date conducted by the ASC ( N = 35 584, N cases = 11 986) identified 102 autism-associated genes ( Fig. 3 ), many of which are expressed during brain development with roles in the regulation of gene expression and neuronal communication (Satterstrom et al., 2020 ). Rare CNVs and SNVs associated with autism have pleiotropic effects, thus increasing the risk for other complex disorders such as schizophrenia, ADHD, ID, and epilepsy (Gudmundsson et al., 2019 ; Satterstrom et al., 2019 , 2020 ).
CNVs can impact one or multiple genes and can occur at common or rare frequencies in a population. All CNVs associated with autism have been rare. Recurrent CNVs are among the most convincing rare inherited risk variations for autism, and have a prevalence of about 3% in affected patients (Bourgeron, 2016 ). In comparison, approximately 4–10% of autistic individuals have de novo deletions or duplications (Bourgeron, 2016 ; Pinto et al., 2010 ; Sebat et al., 2007 ) frequently mapped to established risk loci 1q21.1, 3q29, 7q11.23, 15q11.2-13, and 22q11.2 (Sanders et al., 2015 ). A higher global frequency of de novo CNVs is observed in idiopathic autism cases from simplex families (10%) compared to multiplex families (2%) and controls (1%) (Halladay et al., 2015 ; Itsara et al., 2010 ; Sebat et al., 2007 ). Inherited CNVs can be present in unaffected siblings and parents, suggesting a model of incomplete penetrance dependent on the dosage sensitivity and function of the gene(s) they affect (Vicari et al., 2019 ).
Damaging SNVs include nonsense, frameshift, and splice site mutations (collectively referred to as protein-truncating variants, or PTVs), and missense variants. Rare inherited variants have a smaller average effect size and reduced penetrance compared to de novo pathogenic mutations. Early studies on whole exomes from trios established a key role for de novo germline mutations in autism. Whilst analysis in smaller sample sizes indicated only modest increase in de novo mutation rates in autism cases (Neale et al., 2012 ), the rate rose significantly in excess of expectation as the sample size increased (De Rubeis et al., 2014 ; Iossifov et al., 2014 ). Most recently, the ASC observed a 3.5-fold case enrichment of damaging de novo PTVs and a 2.1-fold enrichment for damaging de novo missense variants (Satterstrom et al., 2020 ), concluding that all exome de novo SNVs explain 1.92% of the variance in autism liability (Satterstrom et al., 2020 ) ( Fig. 2 a ).
Comparatively, the ASC discovered a 1.2-fold enrichment of rare inherited damaging PTVs in cases compared to unaffected siblings (Satterstrom et al., 2020 ). Similarly, recent whole-genome analysis found no excess of rare inherited SNVs, and no difference in the overall rate of these variants in affected subjects compared to unaffected siblings (Ruzzo et al., 2019 ).
New advancements
It is estimated that de novo mutations in protein-coding genes contribute to risk in ~30% of simplex autism cases (Yuen et al., 2017 ; Zhou et al., 2019 ). However, recent work has also shown that de novo mutations in non-coding regions of the genome (particularly gene promoters) contribute to autism (An et al., 2018 ; Zhou et al., 2019 ). Adapting machine learning techniques may be key to providing novel neurobiological insights to the genetic influences on autism in the future (An et al., 2018 ; Ruzzo et al., 2019 ; Zhou et al., 2019 ). Additionally, rare tandem repeat expansions in genic regions are more prevalent among autism cases than their unaffected siblings, with a combined contribution of ~2.6% to the risk of autism (Trost et al., 2020 ).
Common and rare variant interplay
The largest component of genetic risk is derived from common variants of additive effect with a smaller contribution from de novo and rare inherited variation ( Fig. 2 a ) (de la Torre-Ubieta, Won, Stein, & Geschwind, 2016 ; Gaugler et al., 2014 ). Notably, KMT2E was implicated in both the latest GWAS (Grove et al., 2019 ) and exome sequencing (Satterstrom et al., 2020 ) analyses. It is hypothesized that common genetic variation in or near the genes associated with autism influences autism risk, although current sample sizes lack the power to detect the convergence of the two (Satterstrom et al., 2020 ).
Whilst higher SNP-heritability is observed in autistic individuals without ID ( Fig. 2 b ), de novo PTVs in constrained genes are enriched in autistic individuals with ID ( Fig. 2 a ). However, the genetic architecture of autism is complex and diverse. For example, common genetic variants also contribute to risk in autistic individuals with ID and in autistic individuals carrying known large-effect de novo variants in constrained genes (Weiner et al., 2017 ). Furthermore, an excess of disruptive de novo variants is also observed in autistic individuals without co-occurring ID compared to non-autistic individuals (Satterstrom et al., 2020 ).
Epigenetics
DNA methylation (DNAm), an epigenetic modification, allows for both genetic and environmental factors to modulate a phenotype (Martin & Fry, 2018 ; Smith et al., 2014 ). DNAm affects gene expression, regulatory elements, chromatin structure, and alters neuronal development, functioning, as well as survival (Kundaje et al., 2015 ; Lou et al., 2014 ; Peters et al., 2015 ; Sharma, Klein, Barboza, Lohdi, & Toth, 2016 ; Yu et al., 2012 ; Zlatanova, Stancheva, & Caiafa, 2004 ). Additionally, putative prenatal environmental risk factors impact the offspring's methylomic landscape (Anderson, Gillespie, Thiele, Ralph, & Ohm, 2018 ; Cardenas et al., 2018 ; Joubert et al., 2016 ), thus providing a plausible molecular mechanism to modulate the neurodevelopmental origins of autism.
Autism Epigenome-Wide Association Study (EWAS) meta-analysis performed in blood from children and adolescents from SEED and SSC cohorts ( N cases = 796, N controls = 858) identified seven differentially methylated positions (DMPs) associated ( p < 10 × 10 −05 ) with autism, five of them also reported to have brain-based autism associations. The associated DMPs annotated to CENPM , FENDRR , SNRNP200 , PGLYRP4 , EZH1 , DIO3 , and CCDC181 genes, with the last site having the largest effect size and the same direction of association with autism across the prefrontal cortex, temporal cortex, and cerebellum (Andrews et al., 2018 ). The study reported moderate enrichment of methylation Quantitative Trait Loci (mQTLs) among the associated findings, suggesting top autism DMPs to be under genetic control (Andrews et al., 2018 ). These findings were further extended by the MINERvA cohort that added 1263 neonatal blood samples to the meta-analysis. The SEED-SSC-MINERvA meta-EWAS identified 45 DMPs, with the top finding showing the consistent direction of association across all three studies annotated to ITLN1 (Hannon et al., 2018 ). The MINERvA sample was also used for EWAS of autism polygenic score, hypothesizing that the polygenic score-associated DNAm variation is less affected by environmental risk factors, which can confound case–control EWAS. Elevated autism polygenic score was associated with two DMPs ( p < 10 × 10 −06 ), annotated to FAM167A / C8orf12 and RP1L1 . Further Bayesian co-localization of mQTL results with autism GWAS findings provided evidence that several SNPs on chromosome 20 are associated both with autism risk and DNAm changes in sites annotated to KIZ , XRN2 , and NKX2-4 (Hannon et al., 2018 ). The mQTL effect of autism risk SNPs was corroborated by an independent study not only in blood, but also in fetal and adult brain tissues, providing additional evidence that autism risk variants can act through DNAm to mediate the risk of the condition (Hammerschlag, Byrne, Bartels, Wray, & Middeldorp, 2020 ).
Since autism risk variants impact an individual's methylomic landscape, studies that investigate DNAm in the carriers of autism risk variants are of interest to provide insight into their epigenetic profiles. A small blood EWAS performed in 52 cases of autism of heterogeneous etiology, nine carriers of 16p11.2del, seven carriers of pathogenic variants in CHD8 , and matched controls found that DNAm patterns did not clearly distinguish autism of the heterogeneous etiology from controls. However, the homogeneous genetically-defined 16p11.2del and CHD8 +/− subgroups were characterized by unique DNAm signatures enriched in biological pathways related to the regulation of central nervous system development, inhibition of postsynaptic membrane potential, and immune system (Siu et al., 2019 ). This finding highlights the need to combine genomic and epigenomic information for a better understanding of the molecular pathophysiology of autism.
It must be noted that a very careful interpretation of findings from peripheral tissues is warranted. DNAm is tissue-specific and therefore EWAS findings obtained from peripheral tissues may not reflect biological processes in the brain. Using the mQTL analytical approach may reduce this challenge, as mQTLs are consistently detected across tissues, developmental stages, and populations (Smith et al., 2014 ). However, not all mQTLs will be detected across tissues and will not necessarily have the same direction of effect (Smith et al., 2014 ). Therefore, it is recommended that all epigenetic findings from peripheral tissues are subjected to replication analyses in human brain samples, additional experimental approaches, and/or Mendelian randomization to strengthen causal inference and explore molecular mediation by DNAm (Walton, Relton, & Caramaschi, 2019 ).
EWASs performed in post-mortem brains have typically been conducted using very small sample sizes, due to limited access to brain tissue (Ladd-Acosta et al., 2014 ; Nardone et al., 2014 ). One of the largest autism EWAS performed in post-mortem brains (43 cases and 38 controls) identified multiple DMPs ( p < 5 × 10 −05 ) associated with autism (31 DMPs in the prefrontal cortex, 52 in the temporal cortex, and two in the cerebellum) (Wong et al., 2019 ), and autism-related co-methylation modules to be significantly enriched for synaptic, neuronal, and immune dysfunction genes (Wong et al., 2019 ). Another post-mortem brain EWAS reported DNAm levels at autism-associated sites to resemble the DNAm states of early fetal brain development (Corley et al., 2019 ). This finding suggests an epigenetic delay in the neurodevelopmental trajectory may be a part of the molecular pathophysiology of autism.
Overall, methylomic studies of autism provide increasing evidence that common genetic risk variants of autism may alter DNAm across tissues, and that the epigenetic dysregulation of neuronal processes can contribute to the development of autism. Stratification of study participants based on their genetic risk variants may provide deeper insight into the role of aberrant epigenetic regulation in subgroups within autism.
Transcriptomics
Transcriptomics of peripheral tissues.
Gene expression plays a key role in determining the functional consequences of genes and identifying genetic networks underlying a disorder. One of the earliest studies on genome-wide transcriptome (Nishimura et al., 2007 ) investigated blood-derived lymphoblastoid cells gene expression from a small set of males with autism ( N = 15) and controls. Hierarchical clustering on microarray expression data followed by differentially expressed gene (DEG) analysis revealed a set of dysregulated genes in autism compared to controls. This approach was adopted (Luo et al., 2012 ) to investigate DEGs in a cohort of 244 families with autism probands (index autism case in a family) known to carry de novo pathogenic or variants of unknown significance and discordant sibling carriers of non-pathogenic CNVs. From genome-wide microarray transcriptome data, this study identified significant enrichment of outlier genes that are differentially expressed and reside within the proband rare/ de novo CNVs. Pathway enrichment of these outlier genes identified neural-related pathways, including neuropeptide signaling, synaptogenesis, and cell adhesion. Distinct expression changes of these outlier genes were identified in recurrent pathogenic CNVs, i.e. 16p11.2 microdeletions, 16p11.2 microduplications, and 7q11.23 duplications. Recently, multiple independent genome-wide blood-derived transcriptome analysis (Filosi et al., 2020 ; Lombardo et al., 2018 ; Tylee et al., 2017 ) showed the efficiency of detecting dysregulated genes in autism, including aberrant expression patterns of long non-coding RNAs (Sayad, Omrani, Fallah, Taheri, & Ghafouri-Fard, 2019 ).
Transcriptomics of post-mortem brain tissue
Although blood-derived transcriptome can be feasible to study due to easy access to the biological specimen, blood transcriptome results are not necessarily representative of the transcriptional machinery in the brain (GTEx Consortium, 2017 ). Hence, it is extremely hard to establish a causal relationship between blood transcriptional dysregulations and phenotypes in autism. A landmark initiative by Allen Brain Institute to profile human developing brain expression patterns (RNA-seq) from post-mortem tissue enabled neurodevelopmental research to investigate gene expression in the brain (Sunkin et al., 2013 ). Analyzing post-mortem brain tissue, multiple studies identified dysregulation of genes at the level of gene exons impacted by rare/ de novo mutations in autism (Uddin et al., 2014 ; Xiong et al., 2015 ), including high-resolution detection of exon splicing or novel transcript using brain tissue RNA sequencing (RNA-seq). High-resolution RNA-seq enabled autism brain transcriptome analysis on non-coding elements, and independent studies identified an association with long non-coding RNA and enhancer RNA dysregulation (Wang et al., 2015 ; Yao et al., 2015 ; Ziats & Rennert, 2013 ).
Although it is difficult to access post-mortem brain tissue from autistic individuals, studies of whole-genome transcriptome from autism and control brains have revealed significantly disrupted pathways ( Fig. 4 ) related to synaptic connectivity, neurotransmitter, neuron projection and vesicles, and chromatin remodeling pathways (Ayhan & Konopka, 2019 ; Gordon et al., 2019 ; Voineagu et al., 2011 ). Recently, an integrated genomic study also identified from autism brain tissue a component of upregulated immune processes associated with hypomethylation (Ramaswami et al., 2020 ). These reported pathways are in strong accordance with numerous independent autism studies that integrated genetic data with brain transcriptomes (Courchesne, Gazestani, & Lewis, 2020 ; Uddin et al., 2014 ; Yuen et al., 2017 ). A large-scale analysis of brain transcriptome from individuals with autism identified allele-specific expressions of genes that are often found to be impacted by pathogenic de novo mutations (Lee et al., 2019 a ). The majority of the studies are in consensus that genes that are highly active during prenatal brain development are enriched for clinically relevant mutations in autism (Turner et al., 2017 ; Uddin et al., 2014 ; Yuen et al., 2017 ). Recently, a large number (4635) of expression quantitative trait loci were identified that were enriched in prenatal brain-specific regulatory regions comprised of genes with distinct transcriptome modules that are associated with autism (Walker et al., 2019 ).

Most commonly reported three pathways (Ayhan & Konopka, 2019 ; Gordon et al., 2019 ; Voineagu et al., 2011 ) associated with autism. ( a ) The synaptic connectivity and neurotransmitter pathway involves genes (yellow rectangular box) within presynaptic and postsynaptic neurons. Neurotransmitter transport through numerous receptors is an essential function of this pathway; ( b ) the chromatin remodeling pathway involves binding of remodeling complexes that initiate the repositioning (move, eject, or restructure) of nucleosomes that potentially can disrupt gene regulation; and ( c ) the neural projection pathway [adapted from Greig, Woodworth, Galazo, Padmanabhan, & Macklis ( 2013 )] involves the projection of neural dendrite into distant regions and the migration of neuronal cells through ventricular (VZ) and subventricular zones (SVZ) into the different cortical layers (I-VI).
Single-cell transcriptomics
Recent advancement of single-cell transcriptomics enables the detection of cell types that are relevant to disorder etiology. A recent case–control study conducted single-cell transcriptomics analysis on 15 autism and 16 control cortical post-mortem brain tissues generating over 100 000 single-cell transcriptomics data (Velmeshev et al., 2019 ). Cell-type analysis revealed dysregulations of a specific group of genes in cortico-cortical projection neurons that correlate with autism severity (Velmeshev et al., 2019 ). Deciphering cell-type identification has future implications, in particular for the implementation of precision medicine. However, single-cell technology is at very early stages of development and computationally it is still very complex to classify cell-type identity.
The emergence of CRISPR/Cas9 genome editing technology can potentially become an effective tool in future therapeutics of genetic conditions associated with autism. Although introducing and reversing DNA mutation is becoming a mature technology within in vitro systems, much work needs to be done for in vivo use of genome editing. Single-cell OMICs is another emerging field that has the potential to decipher developmental (spatio-temporally) brain cell types that are associated with autism. Identifying cell clusters and defining cell identity is a major computational challenge. Artificial intelligence can significantly improve these computational challenges to identify the molecular associations of autism at the single-cell level.
Clinical and therapeutic implications
In some, but not all, best practice clinical guidelines, genetic tests such as fragile X testing, chromosomal microarray, and karyotype testing are part of the standard medical assessment in a diagnostic evaluation of autism to identify potentially etiologically relevant rare genetic variants (Barton et al., 2018 ). The guidelines vary with respect to whether genetic testing is recommended for all people with autism, or based on particular risk factors, such as ID, seizures, or dysmorphic features. The DSM-5 diagnosis of autism includes a specifier for associated genetic conditions (APA, 2013 ). Although genetic test results may not usually have consequences for treatment changes, the results could inform recurrence risk and provide families with access to information about symptoms and prognosis. In the future, gene therapy, CRISPR/Cas9, and genome editing technologies may lead to the gene-specific design of precision medicine for rare syndromic forms of autism (Benger, Kinali, & Mazarakis, 2018 ; Gori et al., 2015 ).
Given that a substantial proportion of the genetic liability to autism is estimated to be explained by the cumulative effect of a large number of common SNPs, polygenic scores have gained traction as potential biomarkers. However, the predictive ability of polygenic scores from the largest autism GWAS to date is too low to be clinically useful. The odds ratio when comparing the top and bottom polygenic score decile groups is only 2.80 (95% CI 2.53–3.10) (Grove et al., 2019 ). Additionally, polygenic scores based on the samples of European ancestry do not translate well in populations with diverse ancestry (Palk, Dalvie, de Vries, Martin, & Stein, 2019 ).
Genetic testing can in the future become useful for informing screening or triaging for diagnostic assessments or identifying who may be more likely to respond to which type of intervention (Wray et al., 2021 ). Genetics may also help identify individuals with autism who are at a high risk of developing co-occurring physical and mental health conditions or likely to benefit from treatments of such conditions. A top research priority for autistic people and their families is addressing co-occurring mental health problems (Autistica, 2016 ), which may sometimes be the primary treatment need as opposed to autism per se . Genomics may also be helpful to repurpose existing treatments and better identify promising treatments. There are active clinical trials to repurpose drugs in autism (Hong & Erickson, 2019 ). Moreover, genetics can be used to identify social and environmental mediating and moderating factors (Pingault et al., 2018 ), which could inform interventions to improve the lives of autistic people.
Notably, there are important ethical challenges related to clinical translation of advances in genetics, including concerns about discriminatory use, eugenics concerning prenatal genetic testing, and challenges in interpretation and feedback (Palk et al., 2019 ). People with autism and their families are key stakeholders in genetic studies of autism and essential to include in discussions of how genetic testing should be used.
Conclusions and future directions
Recent large-scale and internationally collaborative investigations have led to a better understanding of the genetic contributions to autism. This includes identifying the first robustly associated common genetic variants with small individual effects (Grove et al., 2019 ) and over 100 genes implicated by rare, mostly de novo , variants of large effects (Sanders et al., 2015 ; Satterstrom et al., 2020 ). These and other findings show that the genetic architecture of autism is complex, diverse, and context-dependent, highlighting a need to study the interplay between different types of genetic variants, identify genetic and non-genetic factors influencing their penetrance, and better map the genetic variants to phenotypic heterogeneity within autism.
Immense collaborative efforts are needed to identify converging and distinct biological mechanisms for autism and subgroups within autism, which can in turn inform treatment (Thapar & Rutter, 2020 ). It is crucial to invest in multidimensional and longitudinal measurements of both core defining traits and associated traits such as language, intellectual, emotional, and behavioral functioning, and to collaboratively establish large omics databases including genomics, epigenomics, transcriptomics, proteomics, and brain connectomics (Searles Quick, Wang, & State, 2020 ). Indeed, large-scale multi-omic investigations are becoming possible in the context of large population-based family cohorts with rich prospective and longitudinal information on environmental exposures and developmental trajectories of different neurodevelopmental traits. Finally, novel methods (Neumeyer, Hemani, & Zeggini, 2020 ) can help investigate causal molecular pathways between genetic variants and autism and autistic traits.
Acknowledgements
We thank the Psychiatric Genomics Consortium, Anders Børglum, and Elise Robinson for their support and advice.
Financial support
Alexandra Havdahl was supported by the South-Eastern Norway Regional Health Authority (#2018059, career grant #2020022) and the Norwegian Research Council (#274611 PI Ted Reichborn-Kjennerud and #288083 PI Espen Røysamb). Maria Niarchou was supported by Autism Speaks (#11680). Anna Starnawska was supported by The Lundbeck Foundation Initiative for Integrative Psychiatric Research, iPSYCH, Denmark (R155-2014-1724). Varun Warrier is supported by the Bowring Research Fellowship (St. Catharine's College, Cambridge), the Templeton World Charity Foundation, Inc., the Autism Research Trust, and the Wellcome Trust. Celia van der Merwe is supported by the Simons Foundation NeuroDev study (#599648) and the NIH R01MH111813 grant.
Conflict of interest

Study of different autism types finds shared mechanism that may respond to drugs
Researchers detect similar disruptions in the neural development of genetic and unexplained autism.
An analysis of how brains with different forms of autism develop has revealed common underlying mechanisms that may respond to existing medications.
For the study, Rutgers Health researchers used a technique called induced pluripotent stem cells to transform the blood cells of people with both genetic and unexplained (or idiopathic) autism spectrum disorder (ASD) into early brain cells called neural precursor cells. As the precursor cells from both groups matured in the lab, defects in a common signaling pathway that controls structural proteins led them to struggle with an important step in cell differentiation, the growth of neurites, and the cell migration needed for proper brain architecture.
Although some cell lines exhibited too much activity in this mTOR pathway, while others exhibited too little, the researchers could correct both problems and spur better cell differentiation with existing drugs that either stimulate or inhibit the activity of mTOR (mechanistic target of rapamycin).
"Cells in a dish are not fully human cells that have developed in a fetus and functioned in a person, but they are a lot closer than mouse cells," said Emanuel DiCicco-Bloom, a professor of neuroscience and cell biology/pediatrics at Robert Wood Johnson Medical School and senior author of the study in eLife.
"This finding is particularly interesting because the process of growing new synaptic spines when people learn things is completely analogous to the processes we observed in the cells we used for this experiment: growing axons and migrating during fetal development," DiCicco-Bloom said. "So even though this experiment mimicked a process you'd see during early to mid-pregnancy, the same process involving structural proteins is happening right now in you and me, which means that if we took cells from people with autism and found this abnormal regulation of mTOR in their cells in a dish, those people might be candidates as adults for mTOR regulating drugs to improve their function."
The visible symptoms of ASD vary widely but typically feature some repetitive behaviors and some impairment in communication and social interaction. The condition's incidence has increased from about 1 in 150 children in 2000 to 1 in 36 children in 2020, according to the
Centers for Disease Control and Prevention. Roughly 10 to 15 percent of people with ASD have genes that are known to elevate their risk for ASD. Other cases are idiopathic, meaning they are unexplained.
Rutgers Health researchers began the study with blood from three unrelated people with idiopathic ASD, ages 4 to 14 years, with the expectation of finding person-specific differences in the processes occurring during development in utero. When the researchers used the pluripotent stem cell technique to transform blood cells into the sort of neuron precursors typically found in fetal brains, they unexpectedly found many similarities, including abnormalities in the mTOR pathway, which regulates cell creation, metabolism, neurite growth, remodeling and destruction, among many other functions.
The researchers then gained access to blood cells from another three patients with ASD caused by a particular genetic abnormality associated with about 1 percent of ASD, deletion of genes on chromosome 16, called 16p11.2 deletion. They performed the same experiment and found the same disruptions in neuron development.
Subsequent analysis showed that the mTOR signaling disruptions in some patients stemmed from excessive amounts of a particular molecule, while the disruptions in others stemmed from insufficient amounts. In either case, the researchers could use existing medications approved for use in other conditions to correct the problem and stimulate normal development.
The study team has already begun a follow-up investigation to see if people with ASD stemming from other genetic causes exhibit similar disruptions in mTOR activity during development. If mTOR signaling disruption proves a common feature of ASD, tests of mTOR function could help clinicians diagnose the condition more accurately and differentiate it from other conditions with similar effects.
"A couple of very rare genetic types of autism have already been linked to the mTOR pathway, but this is the first to connect mTOR with genes in the 16p11.2 area, which does not have mTOR on it, and with three presumably different types of idiopathic autism from three unrelated people," said Smrithi Prem, lead author of the study and a psychiatry resident at Penn Medicine who led the study as an MD/PhD student at Robert Wood Johnson Medical School.
"These findings also echo something that has appeared in studies of other conditions, that not all people with mTOR dysregulation have excessive activation that needs inhibition," Prem said. "There are two kinds of mTOR dysregulation, but most trials we've run on people with mTOR dysregulation have only used inhibitors. Our findings showed that cells from two of the people we studied needed more mTOR, not less, and that may spur trials that give different types of mTOR treatment to different individual patients."
- Brain Tumor
- Immune System
- Child Development
- Learning Disorders
- Disorders and Syndromes
- Alzheimer's disease
- Autistic spectrum
- Mammal classification
- Birth control
- Erectile dysfunction
- Sleep disorder
Story Source:
Materials provided by Rutgers University . Original written by Andrew Smith. Note: Content may be edited for style and length.
Journal Reference :
- Smrithi Prem, Bharati Dev, Cynthia Peng, Monal Mehta, Rohan Alibutud, Robert J Connacher, Madeline St Thomas, Xiaofeng Zhou, Paul Matteson, Jinchuan Xing, James H Millonig, Emanuel DiCicco-Bloom. Dysregulation of mTOR signaling mediates common neurite and migration defects in both idiopathic and 16p11.2 deletion autism neural precursor cells . eLife , 2024; 13 DOI: 10.7554/eLife.82809
Cite This Page :
Explore More
- Australia On Track for Decades-Long Megadroughts
- Speed of Visual Perception Ranges Widely
- 3D Printed Replica of an Adult Human Ear
- Extremely Fast Wound Healing: New Treatment
- Micro-Lisa! Novel Nano-Scale Laser Writing
- Simple Brain-Computer Link: Gaming With Thoughts
- Clinical Reasoning: Chatbot Vs Physicians
- Understanding People Who Can't Visualize
- Illuminating Oxygen's Journey in the Brain
- DNA Study IDs Descendants of George Washington
Trending Topics
Strange & offbeat.
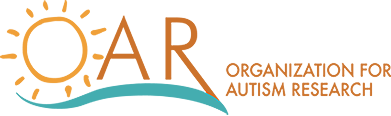
Autism Acceptance Month
This Autism Acceptance Month, OAR remains steadfast in our commitment to championing the autism community through innovative research, educational resources, and unwavering dedication. Join us in celebrating diversity and embracing acceptance this AAM and donate to support our mission today.
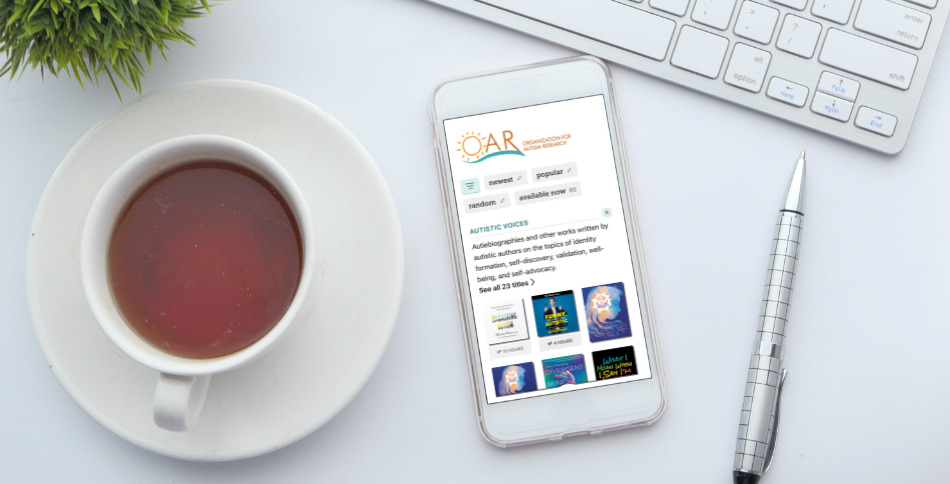
Introducing our Libby Lending Library
Our curated library has free autism-related e-books and audiobooks for parents, self-advocates, professionals, and more. Join us in celebrating autism acceptance by exploring our online collection!

Active for Autism 5K & Kids Dash
Join us in-person or virtually for this fitness & fundraising event in April for Autism Acceptance Month! All proceeds go back to OAR!

Spring Webinar Series on Education
Join us this spring for three webinar events exploring topics in education and supports for teachers and autistic students. Registration is free, so secure your spot today!
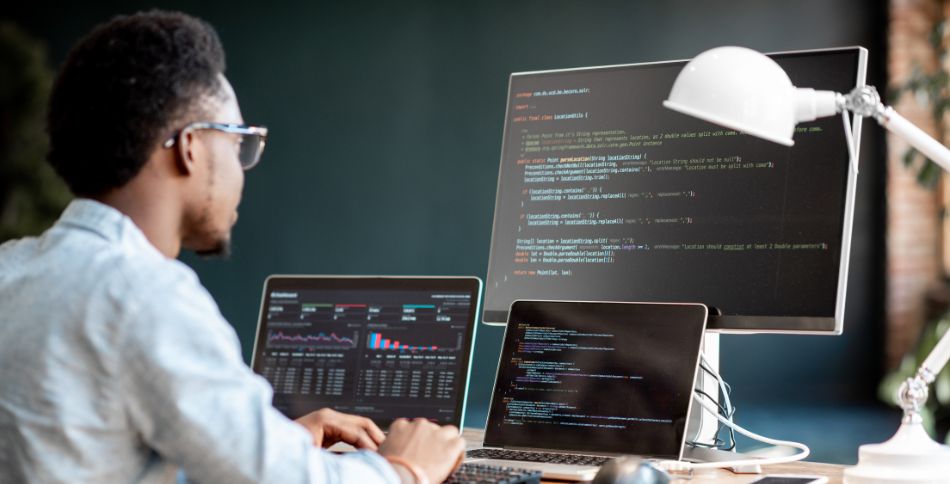
Synchrony Tech Scholarship
Ranging from $500 to $5,000, OAR’s Synchrony Tech Scholarship for autistic adults pursuing a technology-related professional certificate is now open.

Find information you need
We support all members of the autism community
Self-Advocates
Researchers.
The Organization for Autism Research (OAR) was created in December 2001 – the product of the shared vision and unique life experiences of OAR’s seven founders. Led by these parents and grandparents of autistic children and adults, OAR set out to use applied science to answer questions that autistic individuals, their parents, families, teachers, and caregivers confront daily.
No other autism organization has this singular focus.
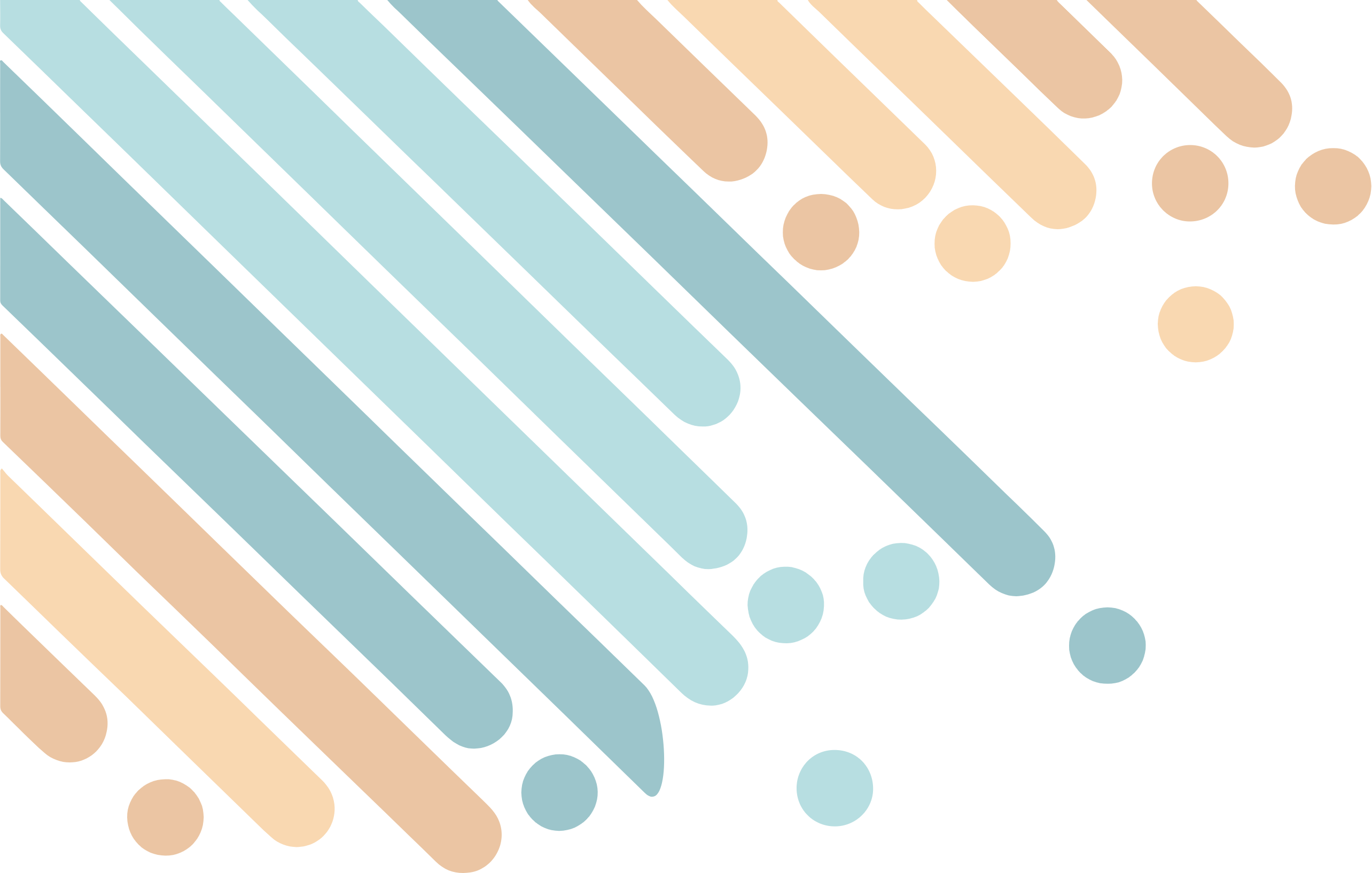
A charity like OAR doesn’t succeed on its own.
We rely on people’s generosity, time, and assistance to help fund research studies, produce and distribute guides and more.
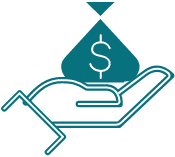
OAR's Impact
OAR is dedicated to improving the lives of everyone impacted by autism by supporting research that produces meaningful and practical results. We keep autistic individuals, families, educators, and professionals informed by offering free, evidence-based resources and information every day.
1 in 36 children
in the U.S. is diagnosed with autism.
Over $4.7 million
in support of autism research.
80% of funds
go directly to research and services.
Over $1.7 million
in scholarships to autism students.
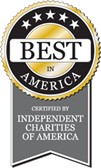
Stay up-to-date with our latest developments, news, and stories.
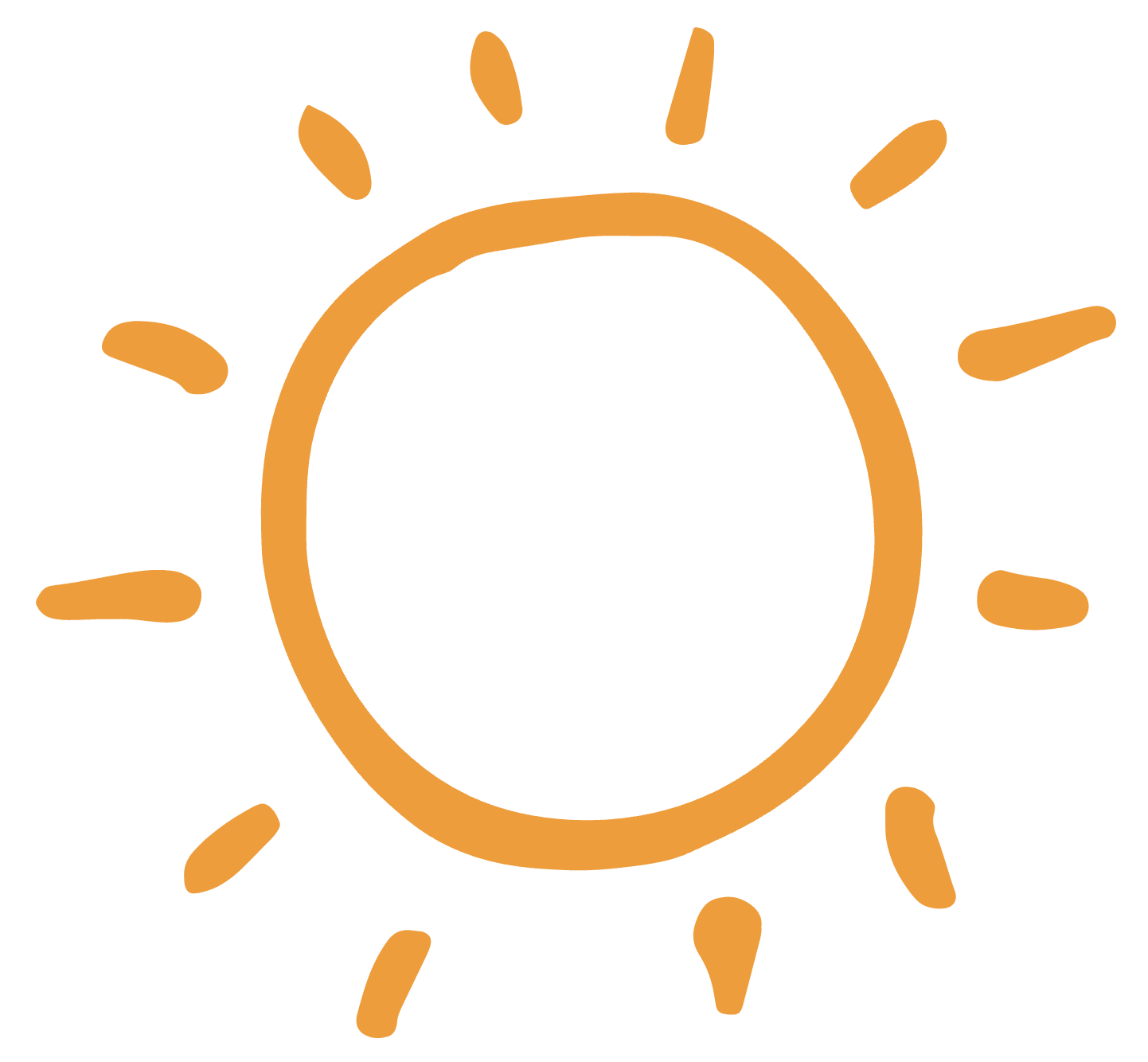
Announcing 2024 Youth Art Contest Winners
Thank you to all the talented artists who participated in OAR’s 2024 Youth Art Contest. With entries from 125 artists across 24 states and five…
April 3, 2024
Read More >
IACC Releases 2019-2020 Research Funding Report
In March, the Interagency Autism Coordinating Committee (IACC) released its 2019-2020 IACC Autism Research Portfolio Analysis Report. The report…
April 2, 2024
OAR Staffer Takes On A New Role
Long-time OAR staff member Rachel Luizza was recently promoted to director of development and marketing. When asked about her new job, she said…
RUN FOR AUTISM Athletes Spring Ahead for OAR
Fifty-nine RUN athletes have raised over $56,000 this spring, dedicating their Chevron Houston Marathon and United Airlines NYC Half Marathon miles to…
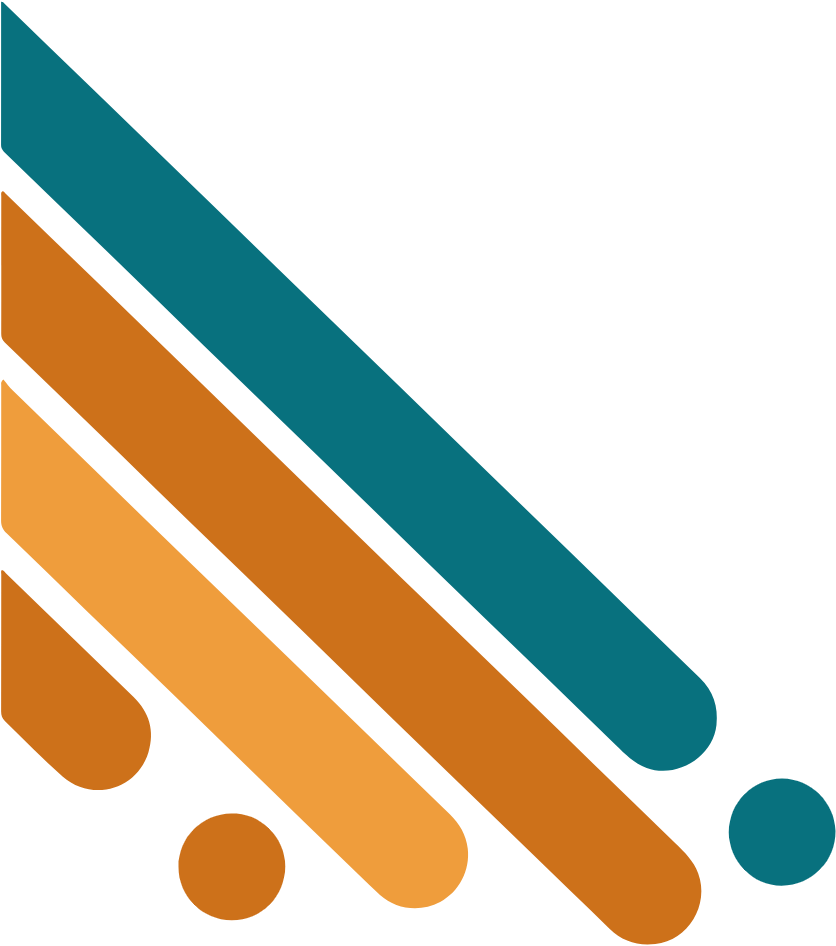
Our Newsletter
You’ll receive periodic updates and articles from OAR

April Is for Awareness and Acceptance: Get Involved! »

Current Research Studies
SARRC’s Research Department is committed to identifying best practices for autism spectrum disorder (ASD) screening, diagnosis, and intervention, but we cannot do it alone. We look to individuals impacted by autism and their families to participate in our current research projects. Our research program utilizes rigorous research methods and is informed by the needs, preferences, and values of the community that we serve. Note: A stipend may be provided to cover the cost of time and travel.
Acadia Study »
The Acadia Study is a 14-week study to learn about irritability associated with autism spectrum disorder (ASD). We are enrolling children and adolescents with ASD ages 5-17 for a clinical study involving an investigational medication. The purpose of this study is to evaluate the effectiveness and safety of the study medication for the treatment of irritability associated with ASD, as well as the efficacy in the treatment of other symptoms associated with ASD, such as social and communication benefits and repetitive behaviors.
Learn more »
EarliPoint™ Diagnostics Research Study »
This study will examine if a new investigational medical device called the EarliPoint™ Evaluation for Autism Spectrum Disorder (ASD), can be used to diagnose autism in children ages 31-84 months. The device detects the presence and severity of ASD and related developmental delays.
The EarliPoint™ Evaluation for ASD (the device) tracks where children are looking as they watch videos on a screen, which shows us how typically developing children and children with autism visually explore the world differently. This study is looking at whether the device can give comparable results to current diagnostic tests performed by clinicians.
First Place Transition Academy Outcomes Evaluation Study »
SARRC is currently enrolling adults with autism ages 18 and older to participate in a study that aims to compare the outcomes of 100 autistic adults who are currently enrolled or who are graduates of the First Place Transition Academy in Phoenix. Participants will help our team improve programs, services and interventions for teens and adults with autism.
Learn more »
IRIS Study »
In the IRIS research study, we are looking to find out whether an investigational drug might improve the symptoms of ASD that often make social interaction challenging. To qualify, participants must be 18 to 45 years of age; have a diagnosis of autism spectrum disorder (ASD) have a relative, housemate, friend, or another study partner to assist during the study, and attend clinic visits.
Leucovorin Study »
SARRC is currently enrolling individuals between the ages of 2.5-5 years with ASD in the Leucovorin Study to assess the efficacy of an investigational natural treatment known as Levoleucovorin in enhancing language and social communication skills in children diagnosed with ASD. The 16-week clinical trial will help our team learn more about language and social communication in children with autism.
MEASURE Study »
The MEASURE Study is a clinical study examining a new investigational medical device, the EarliPoint™ Assessment for autism spectrum disorder in children ages 15-78 months. SARRC is currently enrolling toddlers with and without an autism diagnosis to assess the device’s effectiveness in monitoring a child’s treatment progress.
SPARK for Autism »
The Simons Foundation Autism Research Initiative is offering SPARK—an online, long-term study of genetics and autism. SPARK will collect and analyze genetic samples (saliva) from all participants to help autism researchers learn about genetic and non-genetic causes of autism. SPARK is open to all individuals with a professional diagnosis of autism, as well as their parents. Participation can take place either in your home via a mail-in kit or at SARRC.
- Telemedicine
- Healthcare Professionals
- Go to MyChart
- Find a Doctor
- Make an Appointment
- Cancel an Appointment
- Find a Location
- Visit ED or Urgent Care
- Get Driving Directions
- Refill a Prescription
- Contact Children's
- Pay My Bill
- Estimate My Cost
- Apply for Financial Assistance
- Request My Medical Records
- Find Patient Education
- Refer and Manage a Patient
- Research and Clinical Trials
Autism is a spectrum of disorders with different causes and pathways. So, kids with autism need treatment custom-made for them. To help every child with autism thrive, Seattle Children’s Autism Center researches:
- How autism develops
- How the brain is involved
- How children and teens with autism respond to therapies and services
- How we can improve the quality of life for people with autism spectrum disorder (ASD) and their families
Some studies collect information to help us learn about the causes and effects of autism. Others are called clinical trials. They test new ways to diagnose or treat autism in people. For example, a clinical trial might test a medicine, device or therapy. The goal is to see how well it works and make sure it is safe. We have many studies going on now.
To learn more about how to get involved, call 206-987-7917 or email us . We can let you know of studies that might apply to your child. Your family may be able to take part in research even if you are waiting for an appointment in our clinic.
Our Autism Blog often reports on our research. These projects show some of the many ways we learn about autism and how to better care for children.
SPARK is an autism research study and a growing community of autistic individuals, their families and researchers working together to advance the understanding of autism. Its mission is to improve the lives of people with autism by identifying the causes. This can help inform more effective therapies, treatments, services and supports. The study involves giving a saliva sample for DNA analysis. It is open to people with an autism diagnosis and the parents of children with autism spectrum disorder. SPARK can also connect participants with other autism research studies.
To learn more, see:
- SPARK: Understanding Autism
- After 20 Years, a Mom Finds an Answer (Video 2:52)
A registry is a place where researchers keep information about people who have a condition so they can learn more about it. If you’d like, we can add you to our autism research registry, overseen by Dr. Soo-Jeong Kim . Then, when we begin a new study that may fit your family, we can reach out to tell you about it and you can decide if you want to join.
To find out about joining the registry, call 206-987-7917 or email us .
Biomarkers are ways to measure how the body is working. Dr. Sara Webb works to develop biomarkers that tell how the brain and body work in children with autism and other conditions that affect the nervous system. She uses many ways to get information. These include measuring electrical activity in the brain and heart. She also tests where the eyes look (eye tracking) as a screening tool for toddlers who might have autism.
Webb aims to better understand why some children develop autism. She also wants to improve services for children with social challenges. She directs the Psychophysiology and Behavioral Systems Lab at Seattle Children’s Research Institute.
Seattle Children’s and the University of Washington are part of the Autism Biomarkers Consortium for Clinical Trials .
Four times more boys than girls are diagnosed with autism. Dr. Sara Webb wants to know why. It might be that signs of autism are different in females than in males. Or doctors may miss clues because girls are better at hiding their social struggles. Webb directed the Seattle site for the GENDAAR study. The study’s goal was to understand more about sex differences in brain structure, function, connections and genetics in youth with autism.
Dr. Sara Webb is working with others at the Research in Autism and the Brain Lab at the University of Washington on autism research. They want to learn more about brain signals in people with autism as well as neurotypical people. They use brain imaging, like fMRI (functional magnetic resonance imaging) , to look for differences in brain signals between the 2 groups. Behavioral assessments help them look for links between the signals and behavior.
Most children with 16p11.2 deletion syndrome have symptoms of autism. The syndrome happens when a small piece of chromosome 16 is missing. Seattle Children’s is part of a study to test the medicine arbaclofen in children who have this syndrome. It’s called the L16HTHOUSE study. Researchers are checking whether the medicine helps with development, such as speech, learning and social function.
Dr. Frederick Shic’s team at Seattle Children’s Innovative Technologies Lab makes and studies tools to understand and help children with autism. The tools include robots, video games and technologies that track where kids look and how they process information. These methods:
- Help engage children in learning
- Can help children look at problems in new ways
- Tell us about a child’s strengths and choices
More than 100 genetic changes are linked to autism. Dr. Stephen Smith’s lab works to find out:
- How these changes affect the way the brain works
- If different changes affect the brain in similar ways
Current research focuses on the connections between the brain’s nerve cells. These are called synapses. They control how the brain processes information. The lab’s long-term goal is to group children with autism based on how their genetic changes affect their brains. This would open the door to new treatments for different types of autism. His lab is part of our Center for Integrative Brain Research .
Study after study finds no link between the measles-mumps-rubella (MMR) vaccine and autism. Seattle Children’s doctors work to dispel fears so parents get the lifesaving MMR vaccine for their children. Our former Autism Center director wrote an editorial in the Journal of the American Medical Association about this issue.
How to Take Part in Research Studies
- To learn about research at the Autism Center, call 206-987-7917 or email us .
- See our online listing of research studies and clinical trials .
- Get answers to common questions about taking part in research , such as how we protect your child’s health and safety during research.
Ways to Help
Donations from people like you help researchers explore ideas that could lead to life-changing treatments for kids with autism. Give today .
If you would like an appointment, ask your child’s primary care provider to refer you. Learn more about how to get services at the Autism Center .
Providers, see how to refer a patient .
Also in This Section…
- Contact Us and Location
- How to Get Services
- Meet Your Team
- Treatments and Services
- What to Expect
- Patient and Family Education
- Refer a Patient
- The Autism Blog
Stay Connected
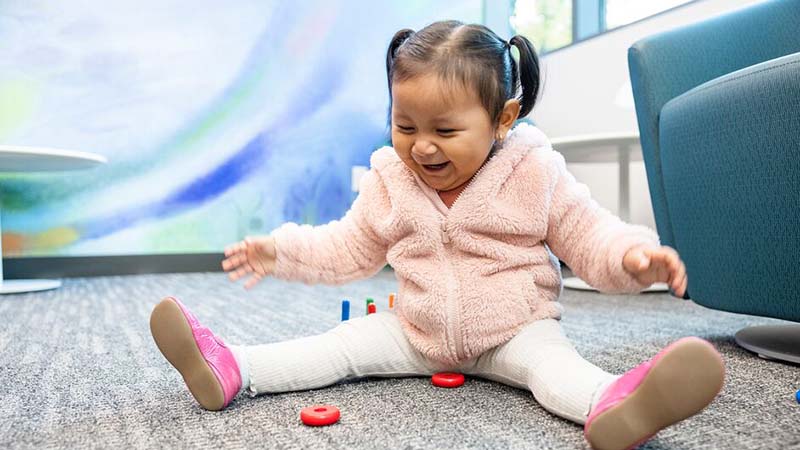
- Read the Autism Blog
- Follow us on Facebook
- Explore resources
- Learn more about the Alyssa Burnett Adult Life Center
Contact Us: 206-987-8080
For Healthcare Professionals
- Manage a Patient
- Provider News
Seattle Children’s complies with applicable federal and other civil rights laws and does not discriminate, exclude people or treat them differently based on race, color, religion (creed), sex, gender identity or expression, sexual orientation, national origin (ancestry), age, disability, or any other status protected by applicable federal, state or local law. Financial assistance for medically necessary services is based on family income and hospital resources and is provided to children under age 21 whose primary residence is in Washington, Alaska, Montana or Idaho.
By clicking “Accept All Cookies,” you agree to the storing of cookies on your device to enhance site navigation, analyze site usage and assist in marketing efforts. For more information, see Website Privacy .

Research Insights: Autistic children’s mental health affected by the quality of their parents’ romantic relationships, mental health — and vice versa

A note on language: There is not a consensus on what terms to use when talking about autism (also called autism spectrum disorder). This interview will use identity-first language (i.e. “autistic child”) as this is often voiced as the preferred term by self-advocates . When working with individuals and families it is always best to ask about their preference.
Brianna Piro-Gambetti , who received her PhD in Human Development & Family Studies (HDFS) in 2023, is the lead author of a recently published study that examined how the mental health of autistic children is connected with the mental health and romantic relationship quality of their parents. She is now a research scientist at the Waisman Center , a research center on the UW–Madison campus that studies human development, developmental disabilities and neurodegenerative diseases.
In this Q&A, Piro-Gambetti talks through the results of her research, which she conducted alongside HDFS professors Sigan Hartley and Lauren Papp and two other co-authors.
What did this study find?
We know that autistic children experience a high rate of mental health conditions like depression, inattention and anxiety relative to neurotypical children. Little is known, though, about how these mental health conditions are related to family dynamics like parent mental health and the quality of the parent-couple relationship. We do know that the family environment is one of the most influential environments for a child and that the well-being of the child is often very closely connected to the well-being of the parents.
With this study, we wanted to understand how autistic children’s mental health is related to parent mental health and parents’ marital or couple relationship across time. Through four study visits conducted over three years, 188 autistic children and their parents came to our lab at UW–Madison and completed questionnaires and interacted with one another while being observed by researchers. We found strong connections between parent mental health and the quality of their parent-couple relationship and the autistic child’s mental health, with effects going in both directions. More specifically, across the three years, increases in depression symptoms in mothers and fathers predicted a decrease in parent-couple relationship satisfaction, and these decreases predicted increases in the autistic child’s mental health conditions. In the other direction, increases in the autistic child’s mental health conditions predicted decreases in parent-couple relationship satisfaction, which in turn, predicted an increase in parents’ depression symptoms, which we saw particularly in fathers.
One interesting pattern we picked up on is that mothers’ depression symptoms appeared to be more directly affected by the child’s mental health conditions, but fathers’ depression symptoms seemed more sensitive to changes in the parent-couple relationship.
Why does this matter?
The focus of autism services tends to be on treating the child, but this study shows it’s crucial to provide support for the whole family. A lot of times when we think of early intervention, there’s a strong focus on behavior modifications for the child and teaching parents how to help their child self-regulate, which is very important. But our study suggests families may also benefit from resources aimed at helping parents with their own mental health and couple relationship needs.
The experiences of family members are interwoven. Parent and child mental health and the quality of the parent-couple relationship are connected in feedback loops across time. By understanding how these dynamics work, we can better tailor interventions to meet the needs of families.
What action should be taken?
Programs and interventions should take a family-wide perspective that addresses the mental health needs of both parents and children as well as the needs of the parent-couple relationship. For example, it could be beneficial to incorporate mental health and parent-couple relationship support into early autism interventions. It’s also important to remember that there’s no one-size-fits-all intervention or program or resource that will work for every autistic child and every family.
What follow-up research is being done?
For this study, we collected mental health data about the child only from their parents, but in future research, it is also important to capture mental health as reported by the autistic child themselves. I also believe collecting these data from teachers is important because it’s a perspective outside of the home.
For our ongoing research, we are looking at other family dynamics, such as the sibling relationship and the quality of the parent-child relationship. We’re trying to explore a variety of familial dynamics in order to understand strategies to promote positive outcomes across different family members.
This study, titled “ Parent-couple satisfaction, parent depression, and child mental health in families with autistic children ,” was published Jan. 10, 2024 in Frontiers in Psychiatry.
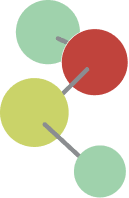
Participate in Research
Scientific discoveries that help families with ASD are only possible through the participation of families in research studies. We know many people want to participate but don’t know where to start.
To address this, ASF created a searchable directory to help you find a study that is right for you. Families, caregivers, service providers and autistic adults can use the directory by searching by age, topic of interest, or geographical location. You can also use the search function to list all studies for a particular topic that you are interested and willing to participate in. Studies that require online participation or a hybrid of online and in person involvement are also included.
Some of these studies will provide financial incentives, others will provide services like supports or interventions and others will share the overall results with you or provide documentation that can help you understand yourself or your family member. Families should reach out directly to the study personnel listed in the contact information to learn more.
For scientists: It is free to include your study in the directory. Please fill out this form If you have a study that you would like to be posted,.
Where are you on your autism journey?
- All News & Stories
- Berry College News
- Berry Stories
- Articles & Guest Blog Posts
- In The News
- Faculty & Staff
- Berry Magazine
- Athletic News
- SHARE A STORY
- Campus Life
- Student Enterprises
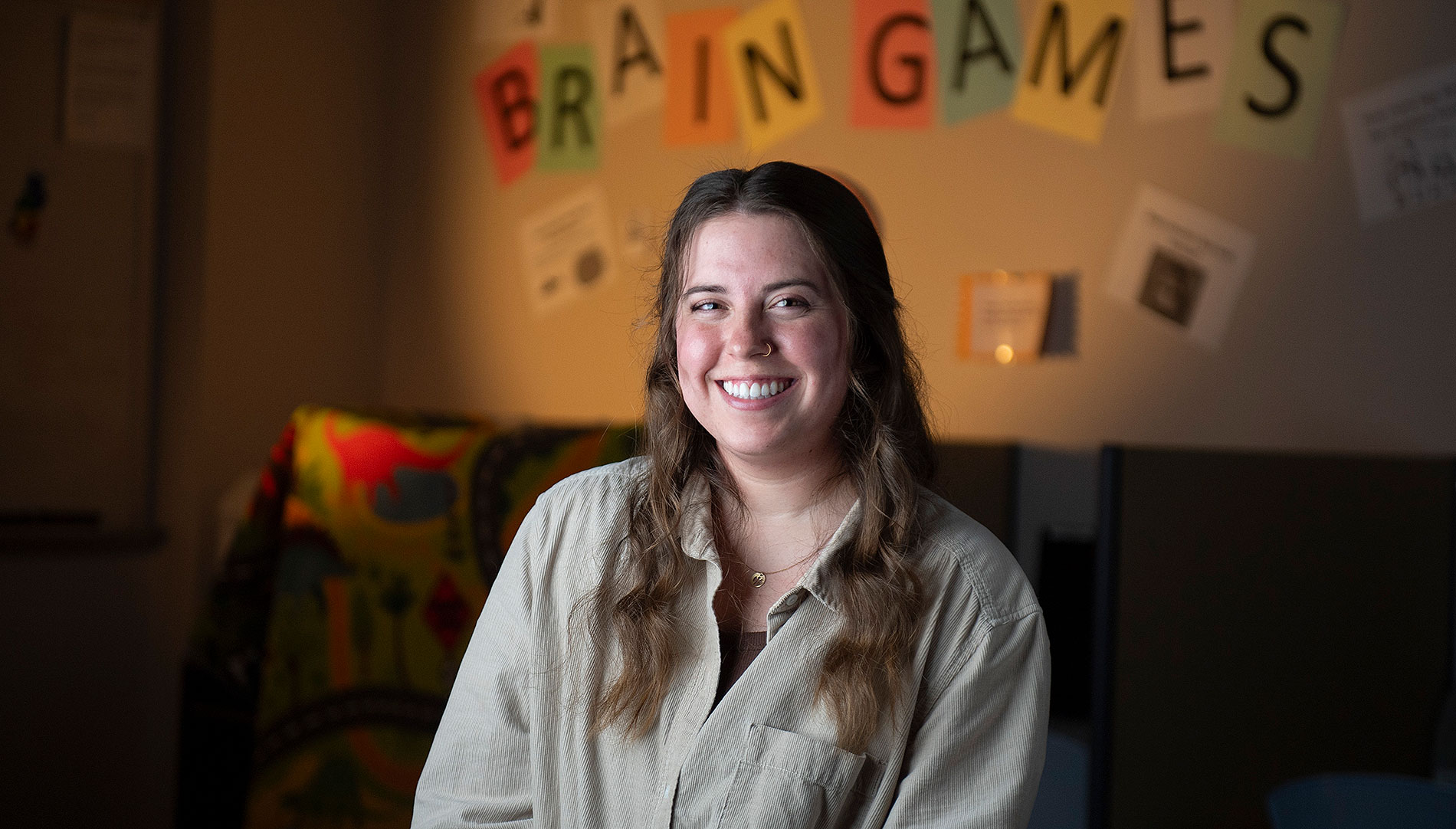
Psychology Major’s Research Empowers Families with Autism
Mackenzie Fowler ’24 has a passion for helping parents of children with autism. As a psychology major with minors in applied behavior analysis (ABA) and art, she plans to work as a board-certified behavior analyst in school environments. Mackenzie’s undergraduate research, study abroad experience and campus job taught her to apply textbook knowledge in the real world and deepened her commitment to the field of psychology.
A required introductory course sparked Mackenzie’s interest in psychology, and a subsequent ABA class introduced her to the science of therapy. She later applied for an internship with a local clinic, C.A.B.S. Autism & Behavior Specialists, where she became a registered behavior technician and began focusing on early intervention with families and parents.
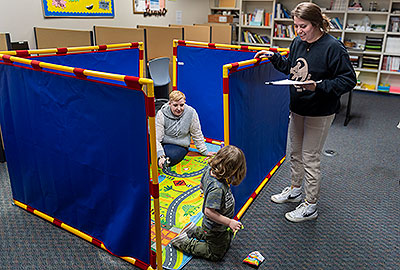
Awarded the psychology department’s George Scholar grant, Mackenzie asked her professor and advisor, Assistant Professor of Psychology Miguel Ampuero, to partner with her on a project. Their research supports parents teaching communication skills to their children with autism. Mackenzie is investigating how much training parents need and how to make it faster and more efficient. Younger psychology students have joined the project, growing undergraduate research opportunities at Berry while giving Mackenzie more mentoring practice.
Her job as the student director of the alumni center also reinforces her supervisory skills: “This role is less focused on discovery and more on professional management experiences such as event planning and building schedules. Here, I’m learning how to manage a work environment and growing professionally in a different way.”
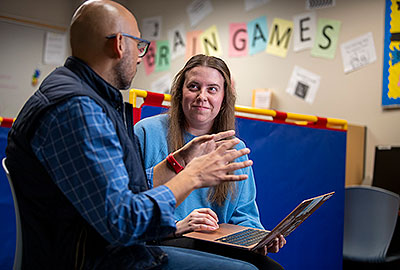
Mackenzie also pursued a summer international program in Peru, saying it was a significant step out of her comfort zone and her most formative college experience. Each year, Berry professors accompany students abroad as they navigate special topics classes and engage in related cultural and professional work. The program coordinators assigned Mackenzie’s group to autism clinics and a special needs school implementing new behavior intervention plans in the classroom.
“If C.A.B.S. helped affirm that I wanted to work in behavior analysis, this trip really solidified it,” she says. “It was amazing to see the basics of the clinics were similar but with cultural differences. It was also neat to see the new program taking shape.”
Recalling how her learning and career possibilities expanded throughout college, Mackenzie is grateful for the ways Ampuero helped shape her future.
“Dr. Ampuero has guided me in so many areas,” says Mackenzie. “He taught several of my classes at Berry, then led me through coursework and practical, real-world experiences in Peru. He also acts as my research advisor, and we plan to publish a paper on our work. He has supported me through every step of my academic and professional decisions in psychology.”
Related Articles
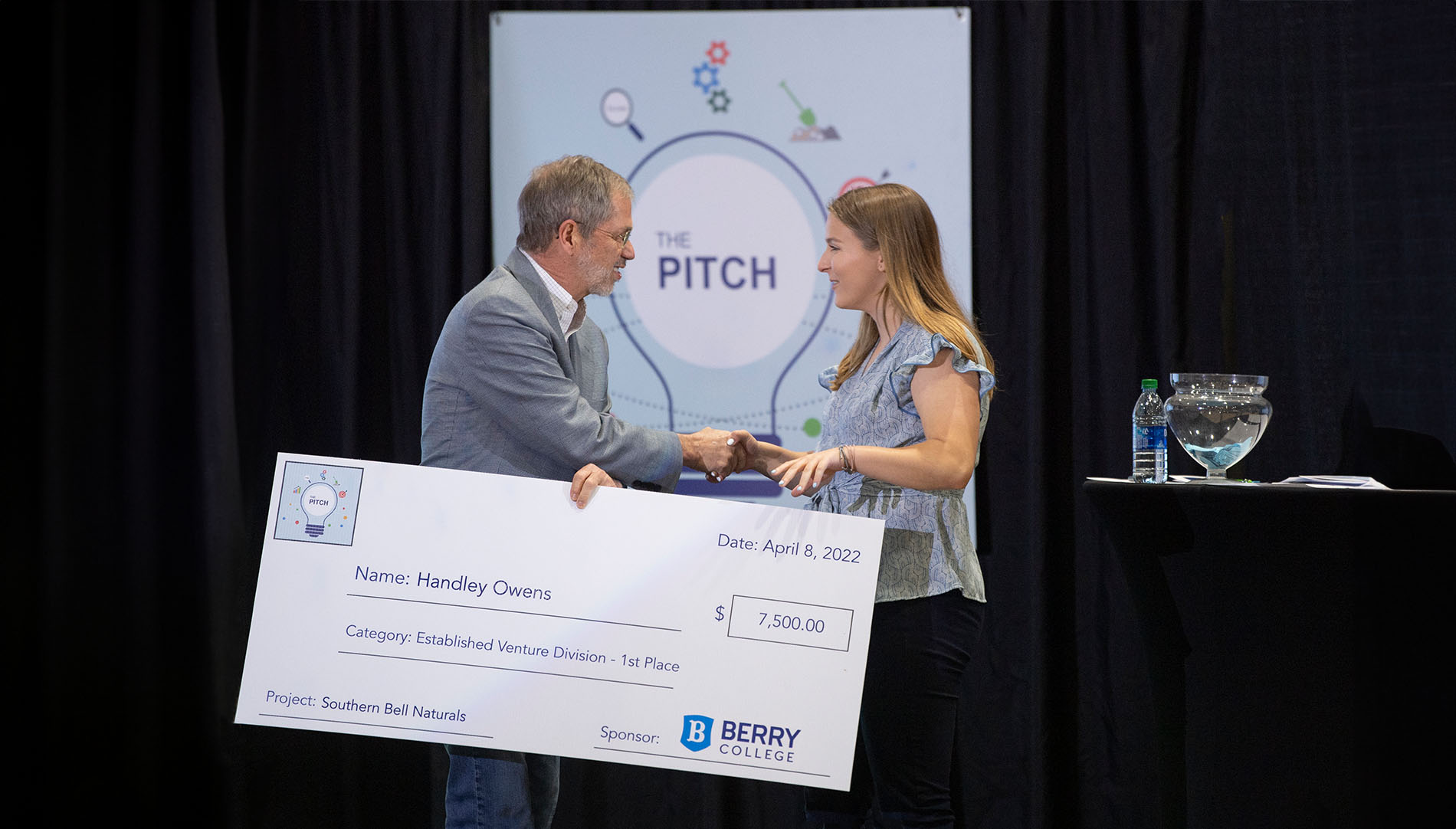
Footer Menu
Puppets could offer valuable support for autistic teenagers
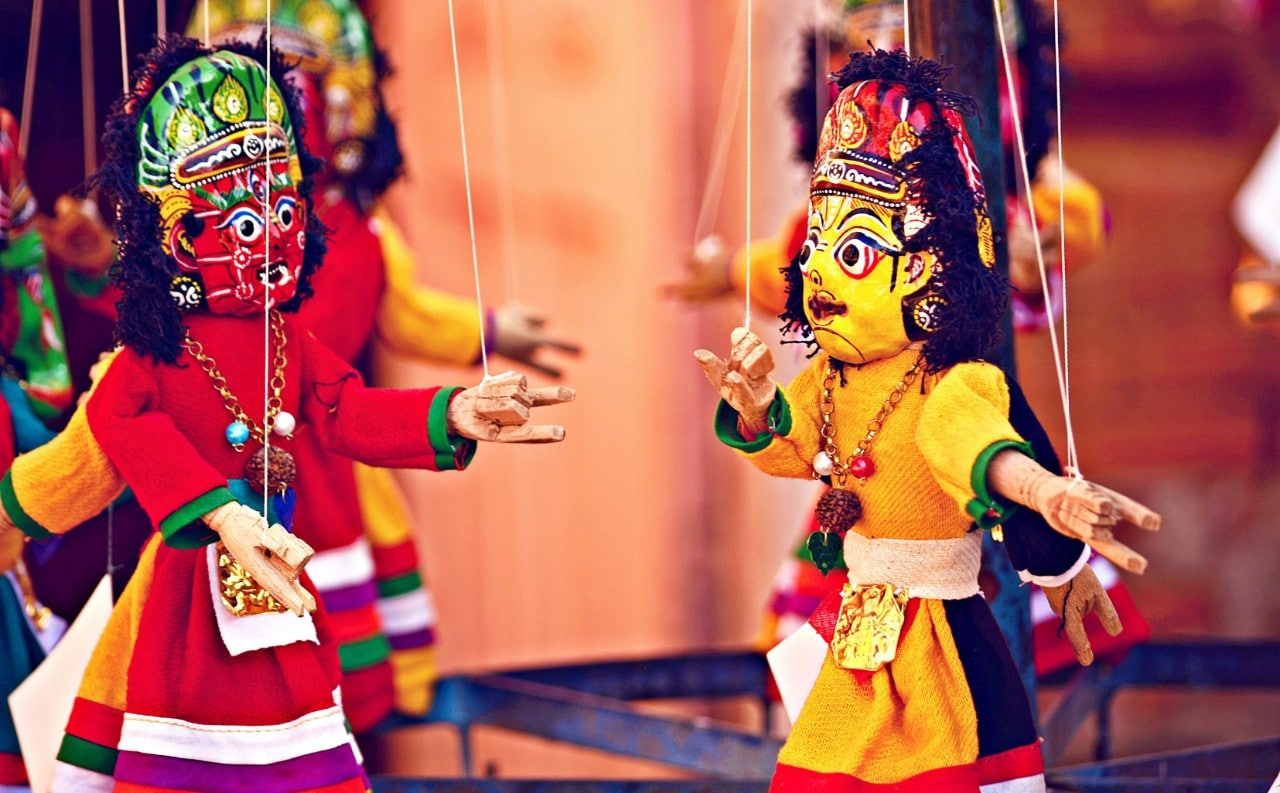
A new study has revealed promising insights into the benefits of using puppets to assist autistic teenagers. The research by Dr Olivia Karaolis, a Special and Inclusive Education Lecturer at the Sydney School of Education and Social Work, explores how puppets used in educational and therapeutic settings may help improve communication, self-expression, and overall well-being among teenagers on the autism spectrum.
Puppets are commonly linked with early childhood education, but Dr Karaolis's study, published in the peer-reviewed journal Youth , demonstrates puppets could be a powerful resource for teenagers, especially those with autism.
“Puppets are more than a toy,” said Dr Karaolis, who has worked extensively with autistic young children, seeing many children speak more frequently and more confidently when a puppet was involved.
“Dramatic play with puppets can serve as a bridge to break down barriers between students and improve communication. The research highlights the potential of puppets to empower autistic teenagers, allowing them to express themselves in ways that respect their strengths and preferences.”
Puppets for teenagers
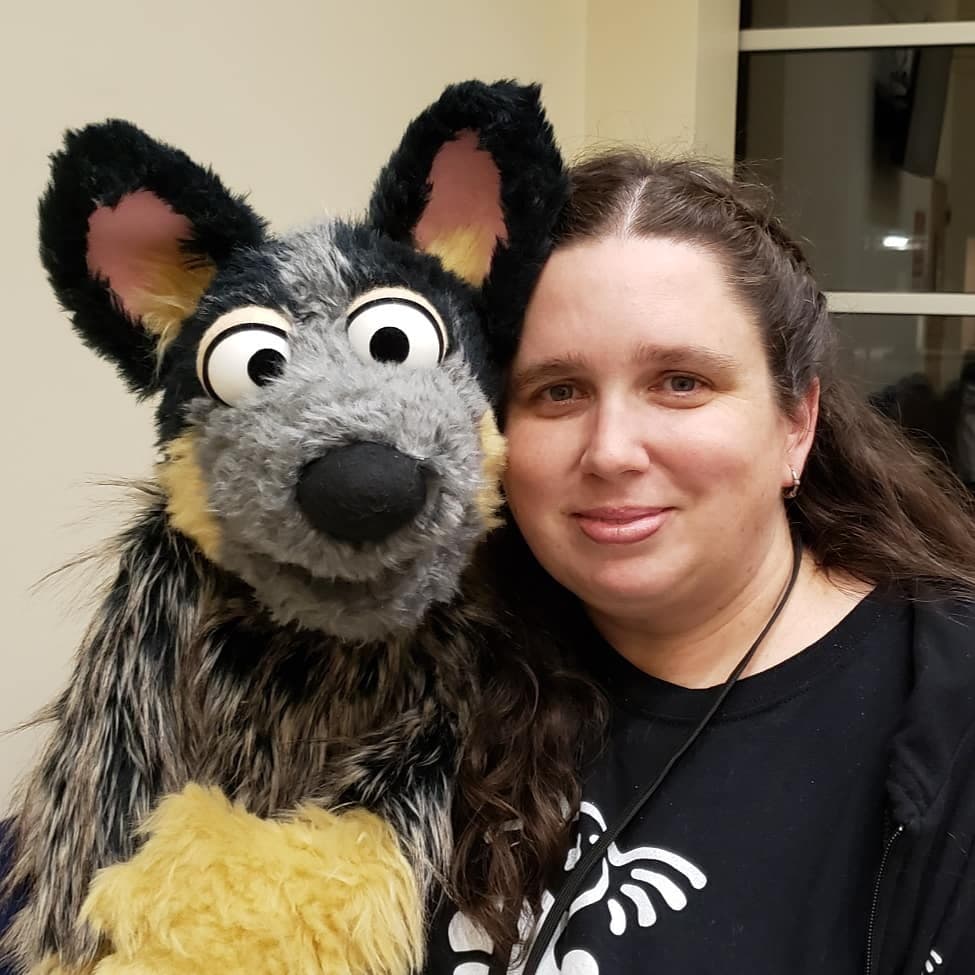
Katherine Hannaford
Ms Katherine Hannaford, a teacher librarian at Macquarie Fields High School in Sydney has been teaching students with autism for 20 years and is an expert in puppet making and play-based learning. She has around 40 puppets on a “tree” in the library at her school.
“Puppets aren’t just for babies and little kids, they are for everyone,” Ms Hannaford said. “I see the Year 7 students come into the library and they might not know anyone yet and they all come from different schools, and the shy ones might start talking to each other, through the puppets. The puppets talk to each other and then when they put down the puppets, the students are more likely to keep talking to each other and make friends.” Older teens working with Ms Hannaford have made the puppets themselves rather than choosing finger puppets or plushie style puppets that are typically given to younger children. Sometimes the puppets are sophisticated marionettes or shadow puppets, and they have helped the students communicate visually in a performance. “I’ve had students performing without fear or self-consciousness because they are behind the puppet,” Ms Hannaford said. “It was the puppet talking and not them.”
For the students on the autism spectrum, the puppets have also been soothing. “Some students calm themselves with touch, and the puppets are very soft. If a student is feeling overwhelmed, sometimes they will take the softer puppets off the tree and sit on a couch and just hold the puppet until they feel better.”
Puppets for high school students
Puppet studies.
The study involved a review of existing literature and peer-reviewed qualitative research. The review included 12 qualitative studies: seven articles that discussed the use of puppets specifically with autistic students in high school settings and five studies that described the use of puppets specifically with autistic teenagers in a range of different therapeutic contexts.
The studies originated from the United Kingdom, the United States, Europe, India and one study from Australia. The studies were selected if they showed evidence of a relationship between puppetry and educational or therapeutic outcomes for teenagers with autism.
Dr Karolis said the study identified a gap in our understanding of how puppets can contribute to the education of autistic teenagers. This gap emphasises the need for further research to explore how puppets can positively impact the lives of autistic teenagers and young people.
“This research introduces new possibilities for educators, therapists, and parents to consider the unique benefits of using puppets in the lives of autistic teenagers,” she said. “As we seek to improve support and opportunities for autistic individuals, the use of puppets as a tool for communication, self-expression, and interaction becomes a compelling avenue worth exploring.”
Tips for secondary school teachers
- Think beyond traditional hand puppets and make and create puppets with your students
- Make puppets from found objects, or puppets from other traditions such as Balinese Puppets, Shadow Puppets or Bunraku
- Use Puppets in the secondary classroom to act out Romeo and Juliet , Macbeth or King Lear . Students can develop an understanding of these characters, their motivations and the themes in the plays through creating a puppet
- Puppets can be used in debates and in whole group discussions and Socratic Circles
- Puppets can be a tool to support design thinking - students can collaborate and respond to a problem or an issue by making a large-scale puppet or puppet performance. Think about the potential of puppets to raise awareness or communicate an important message to the learning community.
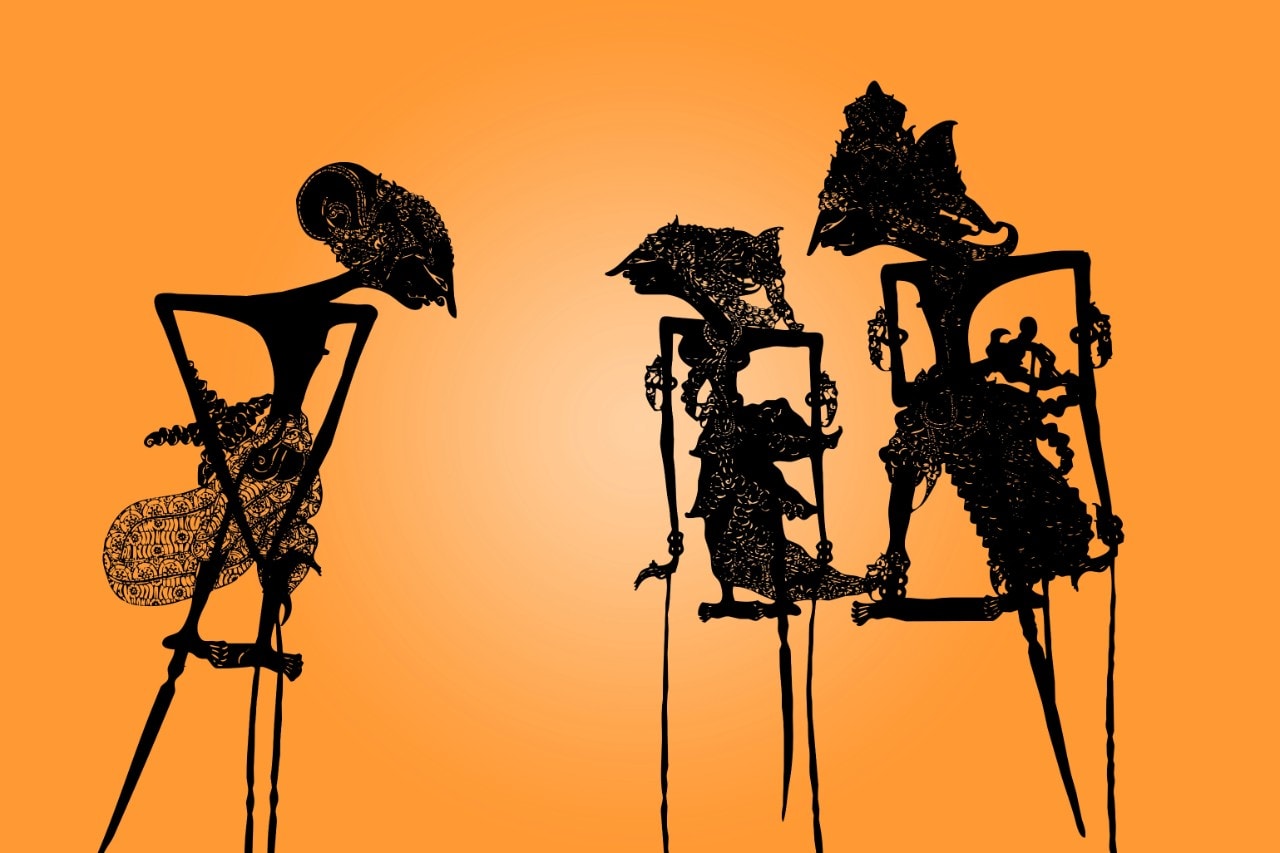
Puppets are used in many cultures: Indonesian shadow puppets. Credit: AdobeStock
Dr Karaolis is a member of the CREATE Centre at University of Sydney (Creativity in Research, Engaging the Arts and Transforming Education, Health and Wellbeing).
Declaration: The research received no external funding. (Hero Photo: AdobeStock)
Media Contact
Elissa blake.
- 0408 565 604
- [email protected]
Related Articles
How to make schools gender inclusive, should taylor swift be taught alongside shakespeare yes, lunar new year 2024: what does the year of the dragon mean.
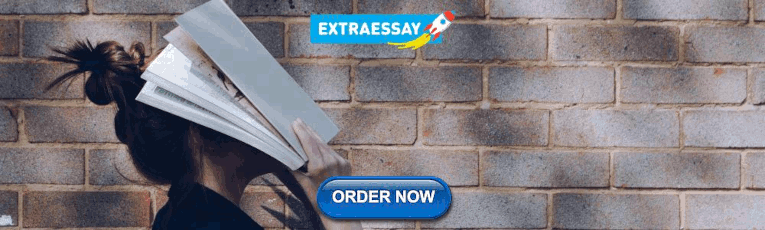
IMAGES
COMMENTS
ARI is a nonprofit organization that supports autism research and education on its causes and treatments. Learn about ARI's latest research grants, webinars, news and resources for autism understanding and support.
This multi-faceted, behavioral intervention can be individualized to improve independence and QoL in adults with Autism Spectrum Disorder (ASD) across the lifespan. The purpose of this study is to confirm a correlation between metal ion dyshomeostasis (low Zn levels, abnormal Zn/Cu ratio, or low Selenium levels) in a North American population ...
If you or your child has a professional diagnosis of autism, Stanford University invites you to learn more about SPARK, a new online research study sponsored by the Simons Foundation Autism Research Initiative. The mission of SPARK is clear: speed up research and advance understanding of autism by creating the nation's largest autism study.
Data were extracted by two independent researchers. Since 2012, 99 estimates from 71 studies were published indicating a global autism prevalence that ranges within and across regions, with a median prevalence of 100/10,000 (range: 1.09/10,000 to 436.0/10,000). The median male‐to‐female ratio was 4.2.
As the definition of autism has subtly changed over the years, so ideas and trends in autism research have waxed and waned. The focus on psychology and behaviour as core descriptive features of autism has, in many respects, guided research and clinical views and opinions about the condition. ... What such genetic studies also served to prove is ...
Autism spectrum disorder (ASD) is a neurodevelopmental disorder characterized by deficits in social communication and the presence of restricted interests and repetitive behaviors ( 1 ). In 2013, the Diagnostic and Statistical Manual of Mental Disorders —5 th edition (DSM-5) was published, updating the diagnostic criteria for ASD from the ...
Autism spectrum disorders are a group of neurodevelopmental disorders that are characterized by impaired social interaction and communication skills, and are often accompanied by other behavioural ...
Through GAPH, Autism Speaks helps underserved communities meet the needs of the world's growing autism population. The goal: To deliver practical and culturally appropriate services that improve lives. We do this through research and partnerships with families, researchers, advocacy groups and governments in more than 70 countries.
In late 2001-early 2002 we received four exciting papers with findings on the genetics of autism that were published together in our March 2002 issue, with an accompanying editorial [2,3,4,5,6 ...
Search for clinical trials on autism spectrum disorder (ASD) sponsored by the National Institute of Child Health and Human Development (NICHD) or other sources. Compare the results and learn more about the studies on ASD.
Autism spectrum disorder (ASD) is a class of neurodevelopmental conditions with a large epidemiological and societal impact worldwide. To date, numerous studies have investigated the associations ...
April 02, 2024. Researchers in the College of Biological Sciences have received a grant to study the role of the cerebellum in autism. "We need a more holistic understanding of the brain circuits that drive this disorder," says Alex Nord, an associate professor of neurobiology, physiology and behavior (NPB), and a researcher at the Center ...
Other avenues of research on autism include investigations into gene variants that could play a role in the development of ASD. A recent study analyzed the DNA of more than 35,584 people worldwide ...
Stanford University researchers are recruiting children with autism to identify brain imaging predictors of benefits from Pivotal Response Treatment (PRT) targeting language abilities. In order to participate in this research study, your child must: Be between the ages of 2 and 4 years. Be able to complete an MRI of the brain during natural ...
JAMA Psychiatry. (2020) 77 (5), 474-483. These three studies were selected as examples of advancements in autism intervention science. According to committee members Connie Kasari, Ph.D., professor of psychiatry at UCLA's David Geffen School of Medicine, and Stelios Georgiades, Ph.D., associate professor of psychiatry and behavioral ...
Studies replicated this year showed that females with autism have a higher burden of rare genetic mutations. In addition, research is demonstrating that females with an autism diagnosis also show a higher level of "common" variations. 29,45 The effect of higher levels of common variation in females extends to even undiagnosed members of ASD-impacted families, demonstrating that females ...
ARI-Funded Research Studies 2022. In 2022, ARI awarded more than $450,000 in grants to fund innovative research that holds realistic promise in impacting the lives of autistic people. At ARI we understand what it means to be different because ARI has always been unique. We have learned a lot over the past 55 years.
Autism spectrum disorder (ASD) is a complex neurodevelopmental condition characterized by social communication challenges and repetitive behaviors. Recent research has increasingly focused on the genetic underpinnings of ASD, with the Neurexin 1 (NRXN1) gene emerging as a key player. This comprehensive systematic review elucidates the contribution of NRXN1 gene variants in the pathophysiology ...
Sex-related differences in the age of autism diagnosis remain poorly understood. In this study, we used electronic health record data to quantify these differences in a large, diverse, academic medical center and determine whether they were associated with the presence of co-occurring neurodevelopmental, psychiatric, and medical conditions in the 2 years preceding autism diagnosis.
For decades, autism research has focused on behavioural, cognitive, social and communication difficulties. These studies highlighted how autistic people face issues with everyday tasks that ...
Thirty years later, twin studies revolutionized the field of autism research (Ronald & Hoekstra, 2011). Twin studies were the first to demonstrate the heritability of autism. In 1977, the first twin-heritability estimate was published, based on a study of 10 dizygotic (DZ) and 11 monozygotic (MZ) pairs (Folstein & Rutter, 1977). Four out of the ...
Study of different autism types finds shared mechanism that may respond to drugs. ScienceDaily . Retrieved March 31, 2024 from www.sciencedaily.com / releases / 2024 / 03 / 240327124553.htm
Research is needed to know whether there are differences in how autistic and non-autistic individuals of different ages process emotions unconsciously. Our study shows that hearing emotionally spoken words unconsciously influenced how non-autistic people understood facial expressions across all age groups, while only non-autistic children were ...
The Organization for Autism Research (OAR) is founded and led by parents and grandparents of autistic children. We are Parent-Led, Science-Based, and Community Focused. ... In November, OAR's Board of Directors authorized funding for eight applied autism research studies in 2024. These new grants, totaling $297,569, bring OAR's total…
The Simons Foundation Autism Research Initiative is offering SPARK—an online, long-term study of genetics and autism. SPARK will collect and analyze genetic samples (saliva) from all participants to help autism researchers learn about genetic and non-genetic causes of autism. SPARK is open to all individuals with a professional diagnosis of ...
To learn about research at the Autism Center, call 206-987-7917 or email us. See our online listing of research studies and clinical trials. Get answers to common questions about taking part in research, such as how we protect your child's health and safety during research.
Brianna Piro-Gambetti, who received her PhD in Human Development & Family Studies (HDFS) in 2023, is the lead author of a recently published study that examined how the mental health of autistic children is connected with the mental health and romantic relationship quality of their parents. She is now a research scientist at the Waisman Center, a research center on the UW-Madison campus that ...
Reset. Scientific discoveries that help families with ASD are only possible through the participation of families in research studies. We know many people want to participate but don't know where to start. To address this, ASF created a searchable directory to help you find a study that is right for you. Families, caregivers, service ...
Mackenzie Fowler '24 has a passion for helping parents of children with autism. As a psychology major with minors in applied behavior analysis (ABA) and art, she plans to work as a board-certified behavior analyst in school environments. Mackenzie's undergraduate research, study abroad experience and campus job taught her to apply textbook ...
A new study has revealed promising insights into the benefits of using puppets to assist autistic teenagers. The research by Dr Olivia Karaolis, a Special and Inclusive Education Lecturer at the Sydney School of Education and Social Work, explores how puppets used in educational and therapeutic settings may help improve communication, self-expression, and overall well-being among teenagers on ...