Thank you for visiting nature.com. You are using a browser version with limited support for CSS. To obtain the best experience, we recommend you use a more up to date browser (or turn off compatibility mode in Internet Explorer). In the meantime, to ensure continued support, we are displaying the site without styles and JavaScript.
- View all journals
- My Account Login
- Explore content
- About the journal
- Publish with us
- Sign up for alerts
- Review Article
- Open access
- Published: 20 September 2022
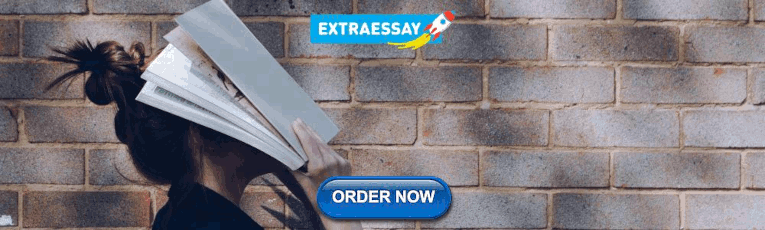
Current understanding of osteoarthritis pathogenesis and relevant new approaches
- Liping Tong 1 ,
- Huan Yu ORCID: orcid.org/0000-0002-1913-5760 1 , 2 ,
- Xingyun Huang 1 , 2 ,
- Jie Shen ORCID: orcid.org/0000-0003-4131-1211 3 ,
- Guozhi Xiao ORCID: orcid.org/0000-0002-4269-2450 4 ,
- Lin Chen 5 ,
- Huaiyu Wang 6 ,
- Lianping Xing ORCID: orcid.org/0000-0002-2588-7766 7 &
- Di Chen 1 , 2
Bone Research volume 10 , Article number: 60 ( 2022 ) Cite this article
17k Accesses
83 Citations
2 Altmetric
Metrics details
- Pathogenesis
Osteoarthritis (OA) is the most common degenerative joint disease that causes painful swelling and permanent damage to the joints in the body. The molecular mechanisms of OA are currently unknown. OA is a heterogeneous disease that affects the entire joint, and multiple tissues are altered during OA development. To better understand the pathological mechanisms of OA, new approaches, methods, and techniques need to be used to understand OA pathogenesis. In this review, we first focus on the epigenetic regulation of OA, with a particular focus on DNA methylation, histone modification, and microRNA regulation, followed by a summary of several key mediators in OA-associated pain. We then introduce several innovative techniques that have been and will continue to be used in the fields of OA and OA-associated pain, such as CRISPR, scRNA sequencing, and lineage tracing. Next, we discuss the timely updates concerning cell death regulation in OA pathology, including pyroptosis, ferroptosis, and autophagy, as well as their individual roles in OA and potential molecular targets in treating OA. Finally, our review highlights new directions on the role of the synovial lymphatic system in OA. An improved understanding of OA pathogenesis will aid in the development of more specific and effective therapeutic interventions for OA.
Similar content being viewed by others
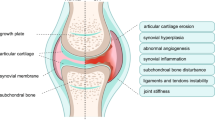
Osteoarthritis: pathogenic signaling pathways and therapeutic targets
Qing Yao, Xiaohao Wu, … Guozhi Xiao
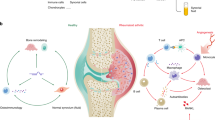
Comprehensive overview of microRNA function in rheumatoid arthritis
Xiaole Peng, Qing Wang, … Dechun Geng
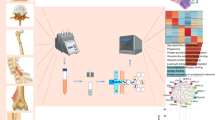
Single-cell RNA sequencing in orthopedic research
Tao Wang, Ling Wang, … Zhiyong Hou
Introduction
Osteoarthritis (OA) is the most common debilitating disease, a leading cause of disability, and is characterized by chronic pain and whole arthropathies such as articular cartilage damage, synovitis, subchondral bone remodeling and osteophyte formation. 1 , 2 , 3 The prevalence of OA is increasing steadily due to the aging population and worldwide obesity epidemic, which brings a great social burden and imposes a major challenge on public health. 4 , 5 , 6 It is estimated that 303 million adults in the world were affected, and in China, approximately 61.2 million people had OA in 2017. 7 Despite the high prevalence, no disease-modifying drugs are currently available. The prescription drugs recommended by international guidelines for OA management merely provide pain relief for symptoms, and the long-term use of these drugs is often associated with significant side effects and toxicities. 8 , 9
OA is a heterogeneous and complicated disease that affects multiple joints, such as the knee, hip, lumbar facet joint, and temporomandibular joint (TMJ). 10 , 11 The risk factors involved in knee and hip OA include genetics, aging, sex (female), race, occupation (physical labor), obesity, hypertension, abnormal joint strength lines, poor muscle strength, high-intensity exercise, and a history of joint injury. 12 , 13 , 14 , 15 , 16 , 17 , 18 These systemic susceptibility factors and local factors can cause abnormalities in signaling pathways and the related regulatory networks of key functional molecules that transmit pain signals and regulate chondrocyte homeostasis, survival and death and ultimately lead to joint pain and pathological cartilage changes in the synovial joint in OA. The pathological mechanisms of OA are currently unknown. Epigenetic regulation is a newly emerging area associated with alterations in catabolic and anabolic gene expression in osteoarthritic chondrocytes. 19 Due to the lack of satisfactory management for OA-associated pain, the mechanisms of OA-associated pain and related signaling pathways remain unclear. 20 , 21 , 22 Recent findings have provided new insights into the roles of new forms of regulated cell death and the synovial lymphatic system in the pathogenesis of OA. 23 , 24 , 25 , 26 To better understand the molecular mechanisms and identify key target(s) for drug discovery and OA treatment, we need to use novel approaches to comprehensively investigate OA mechanisms using newly developed techniques and methodologies. In this review, we will discuss the current understanding of OA pathogenesis and some new approaches, methods, and techniques that have been used in OA research in recent years. First, we focused on the epigenetic regulation of OA, with a particular focus on DNA methylation, histone modification, and microRNA regulation, which have been implicated in OA, and potential epigenome-based therapeutics for OA, followed by a summary of several key mediators in OA-associated pain, including NGF, CGRP, CCL2/CCR2, and TNFα. We then introduced several innovative techniques that have been and will continue to be used in the fields of OA pathology and OA-associated pain, such as CRISPR, scRNA sequencing, and lineage tracing. Next, we discussed cell death regulation in OA pathology, including pyroptosis, ferroptosis, and autophagy, as well as their individual roles in OA and potential molecular targets for treating OA. Finally, our review highlighted new directions on the role of the synovial lymphatic system in OA.
Epigenetic regulation of osteoarthritis
In addition to genetic regulatory mechanisms, the role of epigenetics has recently drawn increasing attention in the regulation of chondrocyte homeostasis, as well as joint integrity and health. Epigenetic regulation in mammalian cells typically involves DNA methylation, histone modification, and noncoding RNA (miRNA and long noncoding RNA) regulation. 27 , 28 Given that epigenetics can govern several signaling pathways simultaneously in a cell-dependent manner, it has been considered a potential therapeutic target to manage OA.
DNA methylation
DNA methylation usually occurs on the cytosine residues of the dinucleotide sequence CpG. The methylation process includes adding a methyl group to the fifth position of cytosine within a CpG dinucleotide to form 5-methylcytosine (5mC), and this process is mainly catalyzed by DNA methyltransferases (DNMTs) (Fig. 1a ). There are three bioactive catalytic DNMTs: DNMT1, DNMT3a, and DNMT3b. 29 , 30 , 31 DNMT1 serves as a maintenance enzyme that binds hemimethylated DNA to maintain the DNA methylation signatures across the genome during cell division and proliferation. DNMT3a and DNMT3b are considered de novo DNMTs since they can create new methylation patterns during development. 29 , 30 , 31 Several studies have established the crucial role of DNMTs in embryonic development. Global ablation the maintenance enzyme DNMT1 leads to embryonic lethality in mice. Compared to DNMT3a, DNMT3b plays a more imperative role in early embryogenesis, and knockout (KO) of DNMT3b causes embryonic lethality with defects in craniofacial and rib cage development, 32 , 33 suggesting a potential regulatory role of DNMT3b in chondrocytes and cartilage development.
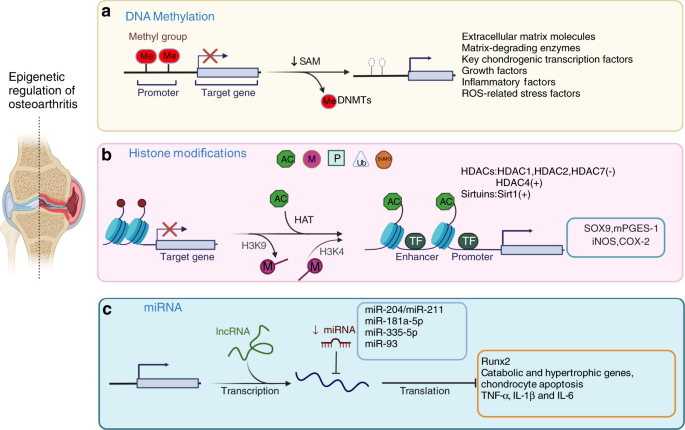
Epigenetic regulation of osteoarthritis. Three types of epigenetic regulation of the molecular pathogenesis of OA. a DNA methylation is catalyzed by DNMTs, and abnormal changes in DNA methylation occur in the promoter regions of related genes and signaling pathways in OA chondrocytes. b Histone modifications included phosphorylation, methylation, acetylation, ubiquitination, and SUMOylation. Histone acetylation is mainly mediated by HATs, and histone deacetylation is usually catalyzed by two types of enzymes: classic histone deacetylases (HDACs) and sirtuins. Increased H3K4 methylation leads to catabolic responses mediated by iNOS and COX-2 expression in human OA chondrocytes. The methylation of H3K9 increases Sox9 and mPGES-1 expression in human OA chondrocytes. c MiR-204/miR-211, miR-181a-5p, miR-335-5p, and miR-93 inhibit Runx2, chondrocyte apoptosis, and the expression of catabolic and hypertrophic genes
In recognition of the roles of DNMTs in chondrocytes, sequencing technology has been used to perform genome-wide DNA methylation analysis has been conducted on healthy individuals and OA patients. 34 , 35 Not surprisingly, several well-known pathways and key factors related to chondrocyte homeostasis have alterations in DNA methylation (5mC) in OA patients. For example, a number of studies have shown abnormal changes in DNA methylation in the promoter regions of related genes in OA chondrocytes, including genes encoding extracellular matrix molecules (e.g., Col2A1, CoL9A1, Col10A1, and Acan), matrix-degrading enzymes (e.g., MMP2, MMP3, MMP9, MMP13, Adamts4/5), and key chondrogenic transcription factors (e.g., Sox9, Sox4, and Runx2). Changes in DNA methylation have also been identified in genes related to signaling pathways, including growth factors [e.g., BMP7, sclerostin and growth differentiation factor 5 (GDF5)], inflammatory factors (e.g., IL-1β, IL-8, C-terminal-binding proteins (CtBP), SOCS2 and LEP), and reactive oxygen species (ROS)-related stress factors [e.g., superoxide dismutase (SOD2) and inducible nitric oxide synthase (iNOS)]. 36 , 37 , 38 , 39 , 40 , 41 DNA methylation is a dynamic process involving DNA methylation and demethylation, and the DNA demethylation signature molecule 5-hydroxy-methylcytosine (5hmC) has been identified in OA chondrocytes. 30 , 42 The 5hmC signature is generated by the ten–eleven translocation cytosine dioxygenases TET1, TET2, and TET3, which catalyze 5mC oxidation and generate 5mC derivatives, resulting in DNA demethylation at cytosine residues. Among the three bioactive TETs, TET1 is one of the major enzymes responsible for DNA methylation in chondrocytes and the generation of 5hmC in OA chondrocytes. Moreover, in addition to the promoter regions of OA-related genes, irregular CpG methylation in enhancer regions in OA chondrocytes has also been reported. 43 , 44 , 45 Although further investigation is needed, the data from these studies indicate that DNA methylation can regulate genes in proximity to enhancers in three-dimensional geometry and in such a way that facilitates gene network regulation associated with chondrocyte function, cartilage maintenance, and OA development.
Since alterations in DNA methylation have been found in OA chondrocytes, most recent studies have been focused on examining the underlying regulatory mechanisms and have mainly focused on DNMTs. Zhu et al. recently demonstrated that the expression of DNMT1 and DNMT3a was elevated in OA cartilage from both OA patients and surgically induced murine OA models. Mechanistically, the increases in DNMT1 and DNMT3a resulted in the hypermethylation of the promoter region of peroxisome proliferator-activated receptor-gamma (PPARγ), leading to suppression of PPARγ expression in OA chondrocytes. More importantly, pharmacological inhibition of DNMT1/DNMT3a by 5-azacitidine could demethylate the PPARγ promoter, restore PPARγ expression, and mitigate the severity of cartilage degeneration in mice, suggesting that the DNMT1/3a PPARγ axis is crucial for OA development. 46 , 47 In contrast, a reduction in DNMT3b expression was observed in human and murine OA cartilage. 48 Specifically, the basal expression level of DNMT3b was higher than that of DNMT1 or DNMT3a in healthy chondrocytes and cartilage tissue; however, DNMT3b expression was significantly decreased in murine OA models and OA patients, at least partially due to an increase in the inflammatory (NF-κB) pathway. Comprehensive genome-wide analysis revealed that DNMT3b deficiency led to the induction of 4-aminobutyrate aminotransferase (Abat) through decreased methylation in the gene promoter, which in turn stimulated tricarboxylic acid (TCA) metabolism and mitochondrial respiration in DNMT3b loss-of-function chondrocytes. However, the increases in Abat expression and mitochondrial metabolism could be restored by the overexpression of DNMT3b in chondrocytes, and importantly, DNMT3b gain-of-function induced a chondroprotective effect to maintain homeostasis and integrity of articular cartilage in the knee joint in mice. 48 Similar to that in the knee joint, the deleterious effect of DNMT3b ablation was also observed in the temporomandibular joint (TMJ). KO of DNMT3b stimulated β-catenin nuclear translocation in TMJ progenitor/stem cells; however, DNMT3b overexpression could normalize Wnt/β-catenin signaling and restore cell homeostasis in vitro. 49 Overall, recent studies showed the critical role of DNMTs in OA development and suggested that DNMTs may be new molecular targets for OA treatment in the clinic.
Histone modification
Histone modification is another type of epigenetic regulation in mammalian cells. Histones are alkaline proteins that are typically found in the nucleus and envelop DNA to assemble nucleosomes. Histone modification includes phosphorylation, methylation, acetylation, ubiquitination, and SUMOylation 50 , 51 (Fig. 1b ). Histone modification usually regulated gene expression by altering chromatin conformation and the ability of transcription factors to access promoter and enhancer regions. 50 , 51 Among these histone modifications, methylation and acetylation are the two mechanisms that are extensively studied in OA. 52 , 53 , 54 , 55 , 56 , 57 , 58 , 59 , 60 , 61
The common site for histone methylation is the lysine (K) residues of histone 3 (H3), and recent studies have examined alterations in the epigenetic status of H3 in human OA chondrocytes. On the one hand, it has been reported that the methylation of H3K9 and H3K27 is increased in the promoter region of the key anabolic gene Sox9 and is associated with Sox9 suppression in human OA chondrocytes. 57 On the other hand, histone methylation has also been shown to be involved in the regulation of the inflammatory response and catabolism in OA development. The Fahmi group evaluated the role of H3K9 and H3K4 methylation in human OA chondrocytes 54 , 55 and found that under inflammatory conditions, the recruitment of the histone demethylase LSD1 was enhanced, leading to a reduction in the methylation level of H3K9 in OA chondrocytes, which in turn stimulated the expression of microsomal prostaglandin E synthase-1 (mPGES-1). Moreover, pharmacological blockade of LSD1 could prevent IL-1β-induced H3K9 demethylation and the induction of mPGES-1 in human chondrocytes. 55 In addition to H3K9 methylation, the Fahmi group investigated H3K4 methylation in human OA chondrocytes. In contrast to H3K9 demethylation, H3K4 methylation was increased by IL-1β in human chondrocytes due to the induced expression of the histone methyltransferase SET‐1A. Increased H3K4 methylation eventually led to catabolic responses mediated by iNOS and COX-2 expression in human OA chondrocytes. 54 , 62 These findings suggest that histone methylation is involved in OA pathogenesis through the regulation of anabolic and catabolic activities in chondrocytes.
Similar to histone methylation, histone acetylation and deacetylation have also been extensively investigated in chondrocytes. 52 , 53 , 56 , 58 , 59 , 60 , 61 In mammalian cells, histone acetylation is mainly mediated by histone acetyltransferases (HATs), and histone deacetylation is usually catalyzed by two types of enzymes: classic histone deacetylases (HDACs) and sirtuins. 63 The expression levels of HDAC1 and HDAC2 are upregulated in OA chondrocytes and are associated with the suppression of anabolic genes, such as Col2a1 and Acan. 52 , 58 , 60 In addition, Huber et al. showed that the expression levels of HDAC1 and HDAC2 were upregulated in OA synovial tissue, indicating an increase in histone deacetylation in joint tissues. 59 HDAC4 has also been extensively studied, although its function is controversial. Lu et al. demonstrated that the expression of HDAC4 was increased in OA cartilage compared to normal cartilage, and a reduction in HDAC4 levels could significantly suppress the expression levels of Mmp1 , Mmp3 , Mmp13 , and Adamts4 / 5 in SW1353 chondrosarcoma cells. 61 However, it has been shown that the expression of HDAC4 is decreased in OA chondrocytes and increases the expression of Runx2 , Mmp13 , Ihh , and Col-X . Therefore, HDAC4 may have chondroprotective effects by inhibiting Runx2 and other OA-related genes. 53 Moreover, correlation studies on Mmp13 and HDAC7 showed that HDAC7 upregulation in OA cartilage was related to enhanced Mmp13 production and ECM degradation. 56 Since histone deacetylation is closely correlated with chondrocyte homeostasis and OA, drugs related to HDACs have also been investigated in vitro and in vivo to prevent cartilage degeneration during OA progression. Inhibitors of HDACs, such as trichostatin (TSA) and vorinostat, and HDAC knockdown by small interfering RNAs have been effective in alleviating ECM degradation and cartilage degeneration during OA progression. 64 , 65 , 66 , 67 , 68 , 69 Furthermore, several siRNAs against HDAC have been approved by the Food and Drug Administration (FDA) in the US for cancer treatment, including vorinostat, romidepsin, valproate and depakote. 66 Further investigation is needed to determine if HDAC siRNAs could be used for OA treatment.
Significant progress has been achieved in clarifying the role of Sirtuin 1 (SIRT1) in OA pathological progression. 70 , 71 , 72 , 73 , 74 Several cellular and animal studies have demonstrated that the SIRT1 protein is found in the nuclei of chondrocytes, as well as in synovial cells. SIRT1 could regulate ECM protein expression in chondrocytes, maintain homeostasis in chondrocytes and cartilage, and play a vital role in inhibiting catabolism, inflammation, oxidative stress, and apoptosis in chondrocytes. SIRT1 expression is decreased in OA chondrocytes. 40 , 70 , 71 , 72 , 73 , 74 Importantly, several studies have demonstrated that the activation of SIRT1 by resveratrol or SRT1720 has chondroprotective effects against cartilage destruction and OA progression. It has been shown that treatment with resveratrol could significantly enhance the gene expression of SIRT1 in chondrocytes, inhibit the IL-1β- or TNFα-induced inflammatory response, and prevent NO-induced apoptosis by regulating Bax and Bcl-2. 75 , 76 , 77 , 78 , 79 In addition to resveratrol, SRT1720, another SIRT1 activator, also showed a protective effect against OA development. Intraperitoneal administration of SRT1720 delayed OA progression in mice at least partially by inhibiting the NF-κB pathway, as well as Mmp13 and Adamts5 expression, in chondrocytes. 80 These studies suggest that SIRT1 activation may serve as an ideal therapeutic strategy for OA treatment.
MicroRNA regulation of osteoarthritis
MicroRNAs (miRNAs) are small, single-stranded RNAs. The average length of a miRNA is 22 nucleotides. MiRNAs negatively modulate target gene expression by binding to the 3′-untranslated region (UTR) of target genes. 81 , 82 , 83 MiRNAs control approximately 50% of the human transcriptome, and human DNA contains more than 45 000 miRNA target sites. 84 , 85 A recent study demonstrated that 142 miRNAs were differentially expressed between damaged and nondamaged OA articular cartilage. To determine the role of miRNA-mediated gene regulation in OA pathology, 238 mRNAs were found to be targeted by differentially expressed miRNAs in OA cartilage using the 2 387 differentially expressed genes as a background. Ten pathways were highly enriched, including the pathways ‘positive regulation of GTPase activity’ and ‘neural development’ associated with nerve growth factor (NGF). 86 In addition, several miRNAs have been shown to play specific roles in OA progression (Fig. 1c ).
Runx2 plays a key role in chondrocyte function. 87 It has been demonstrated that the deletion of one allele of the Runx2 gene in Runx2 global-KO mice or deletion of both alleles of the Runx2 gene in aggrecan-expressing cells in Runx2 conditional-KO mice ( Runx2 Agc1ER ) could significantly delay OA progression caused by destabilization of the medial meniscus (DMM) surgery. 88 , 89 Our lab has identified that miR-204 and miR-211 are two homologous miRNAs that play key roles in the regulation of Runx2 expression in mesenchymal progenitor cells. 90 In the joint tissues of aging mice or OA mouse models, the expression of miR-204/miR-211 was significantly decreased compared to that in young mice or sham-operated mice. Deletion of miR-204 / miR-211 in Prx1 -expressing cells (targeting limb mesenchymal progenitor cells) led to progressive OA development in mice. 91 Significant upregulation of Runx2 protein expression and OA marker genes was observed in miR-204 / miR-211 double-KO mice. Deletion of one allele of Runx2 in a miR-204 / miR-211 double-KO genetic background could significantly reverse the OA phenotype observed in miR-204 / miR-211 double-KO mice. 91 These findings suggest that miR-204/miR-211 have chondroprotective effects during OA development. Zhu et al. found that osteoclast-derived factors coupled with exosomal packaging miRNA play a central role in bone formation through paracrine and juxtacrine mechanisms that affect osteoblast differentiation, suggesting that exosome-mediated delivery of miRNA may be a good strategy to treat OA. 92
MiR-181a-5p is expressed in cartilage in the facet joint (FJ) and is upregulated in human FJ OA and knee OA cartilage. The results were confirmed in the cartilage of rat FJ OA induced by injury and knee traumatic OA. Treatment of rat or mouse chondrocytes with miR-181a-5p antisense oligonucleotides (ASOs) attenuated the expression of marker genes associated with chondrocyte catabolism and apoptosis. MiR-181a-5p ASO treatment reduced cartilage damage, decreased catabolic and hypertrophic gene expression, and inhibited apoptosis and type II collagen degradation in rat FJ OA and mouse knee OA. In addition, the chondroprotective effect of miR-181a-5p ASO was demonstrated in experiments using human OA chondrocytes or ex vivo cartilage explants. 93
MiRNA-335-5p and miR-93 have anti-inflammatory effects. It has been shown that miRNA-335-5p activates AMP-activated protein kinase (AMPK), which is associated with rapamycin (mTOR) signaling and autophagy activation. 94 MiRNA-335-5p inhibits OA chondrocyte inflammation by activating autophagy. 95 MiR-93 attenuates tumor necrosis factor (TNF)-α-, interleukin (IL)-1β- and IL-6-induced inflammatory responses in chondrocytes. 96 These findings demonstrated that miRNAs are closely associated with the regulation of OA development and progression.
Osteoarthritis-associated pain
Pain is the major symptom that prompts individuals with OA to search for medical assistance. Pain is often associated with joint damage in OA patients. However, pain may not always correlate with structural changes in joint tissues. Sometimes there is a separation between joint damage and pain. OA-associated pain predominantly originates from the synovium and subchondral bone of the joint. Except for normal articular cartilage, which lacks nerve innervation, other tissues in the joint, including the meniscus, ligament, tendon, and fat pad, all have abundant sensory nerve innervation and can be the source of pain. Nociceptors are stimulated by mechanical, chemical or thermal noxious stimuli and transduce these noxious inputs into electrical signals, which are transmitted along the dorsal root ganglion and the dorsal horn of the spinal cord. Ultimately, ascending pathways activate the higher brain center, leading to conscious awareness of pain (Fig. 2 ). In contrast to fast pain, the persistent inflammatory microenvironment in the OA joint causes peripheral and central nerve sensitization with typical features of mechanical allodynia and hyperalgesia around the joint. 97 , 98 Despite the unclear mechanism of nerve sensitization, joint replacement can reverse this paresthesia, suggesting that the central nervous system is highly plastic and that peripheral stimulation may be a major intrinsic factor driving central nerve sensitization. 99 A better understanding of the mechanisms of OA-associated pain will help in the diagnosis and treatment of OA.
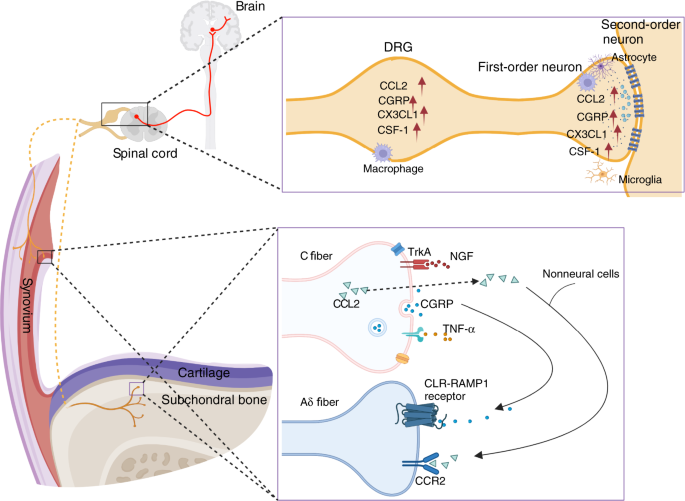
The NGF, CGRP, CCL2/CCR2, and TNFα signaling pathways in OA-associated pain. NGF acts on its receptor TrkA and mediates pain transmission. CGRP is released from C-fiber terminals and binds with calcitonin receptor-like receptor (CLR) and receptor activity-modifying protein 1 (RAMP1) on adjacent Aδ nerve fibers. High expression of chemokines, such as CCL2 and CX3CL1, and other immune mediators, such as colony-stimulating factor 1 (CSF-1) and CGRP, was detected in the DRG and dorsal horn of the spinal cord
Nerve growth factor (NGF) was first identified because it induced the growth of nerves. 100 We now know that NGF is also associated with intractable pain. NGF and its receptor tropomyosin receptor kinase A (TrkA) are considered new targets for treating OA-associated pain. 101 Anti-NGF antibodies and TrkA inhibitors have been developed to suppress NGF/TrkA signaling. 102 Interestingly, local anesthetics can inhibit TrkA. 103 Fasinumab and tanezumab are humanized monoclonal antibodies against NGF. Patients with symptomatic knee or hip OA were administered gradient doses of fasinumab (1, 3, 6, or 9 mg) in a phase III clinical study. 104 The results demonstrated that all doses of fasinumab achieved clinically important relief of pain compared with the placebo at 16 weeks. Destructive arthropathy was reported in one patient who received 6 mg fasinumab. The latest phase III clinical study with a 24-week follow-up period showed that tanezumab (5 and 2.5 mg) significantly improved WOMAC pain and function and Patient’s Global Assessment of OA with a 2.8% incidence rate of rapidly progressive OA in the tanezumab 5 mg and 1.4% in tanezumab 2.5 mg groups. 105 Therefore, the underlying mechanisms of NGF-induced rapidly progressive OA should be carefully and thoroughly examined. It has been reported that increases in the angiogenic factor VEGF, the axonal promoting factor NGF/TrkA and sensory neuronal distribution in individuals with FJ OA and low back pain (LBP) have been detected. These findings were associated with high expression levels of inflammatory cytokines, pain-related molecules, and cartilage-degrading enzymes in the degenerative cartilage of FJ. 106 Seidel et al. reported that NGF was predominantly distributed in damaged cartilaginous tissues (80%) and in bone marrow (20%) in FJ OA, which was distinct from that in the subchondral bone of knee OA. 107 NGF and downstream substance P are associated with cartilage pathology in FJ OA. Despite the high risk of joint damage in knee OA, NGF inhibitors have demonstrated good efficacy in treating LBP. A phase III clinical study of NGF antagonists in the treatment of chronic LBP showed that fasinumab improved both LBP and function, and the adverse effect of rapidly progressive OA only occurred in subjects with accompanying peripheral OA. 108
Calcitonin gene-related peptide (CGRP) is expressed in primary afferent nociceptive unmyelinated C-fibers and is a critical factor in migraine pain. 109 CGRP is expressed in nociceptive C-fibers. CGRP is released from C-fiber terminals and sensitizes adjacent Aδ nerve fibers. 110 Aδ nerve fibers express calcitonin receptor-like receptor (CLR) and receptor activity-modifying protein 1 (RAMP1). These findings are consistent with recent observations that the anti-CGRP antibody fremanezumab blocks induced firing of Aδ but not C-fibers. 111 CGRP receptors were also found in vascular smooth muscle cells and joint tissues innervated by CGRP-positive nerve fibers. 110 Although the source of OA-associated pain has not been fully defined, the detection of substance P (SP) and CGRP fibers in the synovial tissues in mice suggests that synovial tissue may be the major source of pain during OA development. 112 In animal studies, intra-articular injection of CGRP caused mechanical allodynia in naïve mice, and CGRP receptor antagonist and CGRP neutralizing antibody treatment alleviated OA-associated pain. 113 In OA patients, serum CGRP levels and the density of CGRP-positive nerve fibers were highly correlated with OA-associated pain symptoms and disease severity. 114 However, a phase II clinical trial showed that galcanezumab, a CGRP monoclonal antibody, failed to relieve OA-associated pain. 115 Interestingly, accumulating evidence has shown sexual dimorphism in chronic pain. 116 , 117 Recently, Uchida et al. demonstrated that the expression of CGRP and its receptor RAMP1 in the OA synovium was markedly increased in women compared to men, and CGRP expression was positively correlated with pain severity in females but not in males. 118 These findings highlight the different pain mechanisms in males and females with OA. Future studies should determine the role of CGRP signaling in OA-associated pain in females.
The development of pain behavior is a complicated process involving multiple signaling steps, including changes in the expression and production of key mediators of pain in peripheral organs and the expression of pain mediators in the sensory neurons of the dorsal root ganglia (DRG). 119 , 120 Some of this evidence was observed in nerve injury animal models. 121 It has been shown that chemokines and chemokine receptors, especially chemokine (C-C motif) ligand 2 (CCL2) and its receptor chemokine (C-C motif) receptor 2 (CCR2) mediate pain behavior in the DRG and spinal cord. 119 , 122 The role of CCL2/CCR2 signaling in pain mediation during OA development was determined using the DMM OA mouse model. Malfait’s group performed longitudinal studies to monitor pain behaviors and associated molecular mechanisms in the sensory neurons that innervate the knee and demonstrated the role of CCL2/CCR2 in the mediation of pain behavior during OA development. 123
It is well documented that chemokine–chemokine receptor axes can excite DRG nociceptors by activating molecules such as TRP, and sodium channels have been shown to modulate monocyte/macrophage recruitment in multiple inflammatory diseases. 119 , 124 However, the underlying cellular and molecular mechanisms of CCL2/CCR2 signaling in OA-associated pain remain poorly understood. Raghu et al. found that CCL2- and CCR2-deficient mice exhibited reduced OA pathology and low local monocyte/macrophage infiltration in joints. 125 Blocking CCL2/CCR2 signaling with bindarit, a CCL2 synthesis inhibitor, and RS-504393, a CCR2 antagonist, markedly reduced macrophage accumulation and improved synovitis and cartilage lesions in mouse OA. A recent study demonstrated that CCL2/CCR2 signaling caused knee hyperalgesia through direct activation of CCR2 on sensory afferents in peripheral joints. 126 Therefore, CCL2/CCR2 signaling involves both peripheral and central mechanisms that contribute to OA-associated pain. Targeting CCL2/CCR2 signaling in the local joint and in the innervating dorsal root ganglia, as well as the dorsal horn, are potential strategies for treating OA-associated pain.
Inflammation can be a major cause of joint damage and OA-associated pain. Although central nociceptive pathways are involved in OA-associated pain, the interaction of the immune system with nociceptive neurons is critical for inflammatory pain. Following joint damage and peripheral nerve injuries during OA development, neurons release chemokines, such as CCL2 and CX3CL1, and other immune mediators, such as colony-stimulating factor 1 (CSF-1). 127 , 128 , 129 These factors activate microglia and astrocytes, which can release proinflammatory cytokines, including TNF-α, IL-1β, and IL-6, the chemokine CCL2, excitatory amino acids (EAAs), and nitric oxide (NO). 128 , 130 , 131 , 132 Neurons in the dorsal horn of the spinal cord express receptors for many of these inflammatory factors and cytokines, including TNF-α, IL-1β, IL-6, and IL-17. 133 , 134 Activating neuronal cytokine receptors could modify neuronal function. For example, it has been shown that TNF-α and IL-1β promote excitatory synaptic transmission and inhibit inhibitory synaptic transmission in neurons in the spinal cord. 135 , 136
Previous studies have demonstrated that TNF-α is a major proinflammatory cytokine and is closely associated with OA pathogenesis; however, clinical trials of patients with hand OA did not achieve good results. 137 In a multicenter study, patients with inflammatory hand OA that flared after NSAID washout were enrolled for anti-TNF-α therapy. Etanercept did not show notable pain relief compared with placebo after 24 weeks of treatment in erosive OA patients. 138 In the HUMOR trial, subcutaneous injection of adalimumab did not improve VAS pain scores or OA-related pathology, such as synovitis and bone marrow lesions, in patients with erosive hand OA. 139 TNF-α has two high-affinity and specific receptors: TNFR1 and TNFR2. These two receptors are differentially expressed and seem to have distinct functions. TNFR1 is expressed by nearly all cell types and primarily mediates inflammation, while TNFR2 is expressed by certain cells, such as neural cells and vascular endothelial cells, and inhibits inflammation in inflammatory arthritis and neurodegenerative diseases, as well as cardiac diseases. A recent study demonstrated that TNFR2 is also expressed in chondrocytes and that the activation of progranulin (PGRN)/TNFR2/14-3-3ε signaling in chondrocytes alleviates OA-associated pain and protects against OA. 140 These findings suggest that TNFR2 signaling is a promising target for treating OA-associated pain and pathology (Fig. 2 ).
CRISPR technology
The ability to manipulate genetic information is critical for studying gene function and revealing biological mechanisms. Advances in genome and transcriptome engineering technologies have sparked a new revolution for life science research in the past decade. 141 CRISPR/Cas systems are derived from the prokaryote adaptive immune system that protects bacteria and archaea against invading genetic materials. 142 These systems consist of clustered regularly interspaced short palindromic repeats (CRISPR) and multiple genes encoding CRISPR-associated (Cas) proteins that adjoin the CRISPR array. 143 A diverse range of CRISPR/Cas systems has been used to target and modulate nucleic acids. Whole CRISPR/Cas systems rely on CRISPR RNA (crRNA) or on a single guide RNA (sgRNA) for target recognition. The spacer part of the crRNA or sgRNA hybridizes with the targeting sequence that is upstream of the protospacer adjacent motif (PAM) or protospacer flanking sequence (PFS), and then the Cas cleaves the target DNA or RNA. 144 , 145 Thus, by designing crRNA or sgRNA containing proper spacer sequences, CRISPR/Cas systems can achieve cleavage at any locus of interest next to a PAM or a PFS.
Of the current emergence genome editing tools, the most widely used is the class of RNA-guided endonucleases known as Cas9 (Fig. 3a ). Specifically, Streptococcus pyogenes Cas9 (SpCas9) was the first to be reprogrammed for genome editing in eukaryotic cells, and it remains the most commonly used Cas9. 146 Cas9 generates DNA double-strand breaks (DSBs) that activate the DNA damage response and induce repair by the endogenous pathways: nonhomologous end joining (NHEJ) or homologous directed repair (HDR). 147 NHEJ is an error-prone repair process that leads to the generation of small insertions or deletions (Indels). 148 Thus, it is useful for silencing gene expression by disrupting the protein reading frame. 149 HDR is an error-free repair process that corrects pathogenic mutations by using a repair DNA template to stimulate homologous recombination. HDR can be used to introduce the desired change by providing donor DNA that contains a homology sequence to the fractured target site, such as gene site-directed mutation or the insertion of a larger fragment of DNA. 150 It is important to note that NHEJ is markedly more active than HDR. Therefore, increasing the efficiency of HDR following Cas9-mediated DSBs is widely pursued to fully examine the potential of genome editing to introduce accurate genomic modification. 151 , 152
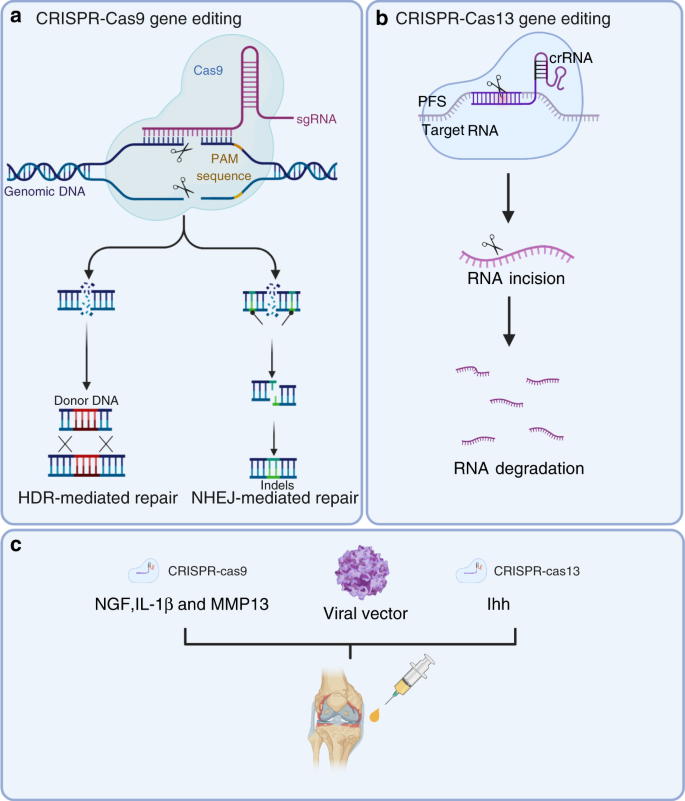
CRISPR/Cas systems have been used for gene editing and treating OA. a Cas9 generates DNA double-strand breaks that activate the DNA damage response and induce repair through various endogenous pathways: nonhomologous end joining (NHEJ) or homologous directed repair (HDR). b Cas13 cuts the target RNA via intrinsic RNase activity. c CRISPR–Cas9-mediated NGF, IL-1β, and MMP13 deletion and CRISPR–Cas13-mediated ihh knockout were used for OA treatment
CRISPR/Cas9 technology has been widely used in the construction of plant, animal, and cellular models. 153 , 154 , 155 A representative example of this is the generation of osteocalcin-knockout rats. 156 The osteocalcin-null rat models showed increased trabecular bone and improved biomechanics. IL-1β is a proinflammatory cytokine that induces the expression of many genes related to OA, such as tumor necrosis factor-alpha (TNF-α). 157 It is interesting to note that exposure of human articular chondrocytes (hAC) to TNF-α leads to increased levels of IL-1β expression. 158 Karlsen and colleagues silenced the IL-1β cytokine receptor (IL1-R1) and simultaneously inserted a puromycin-resistance gene into hACs with CRISPR/Cas9 technology. In their study, unedited cell lines showed increased inflammation when exposed to recombinant IL-1β. Conversely, the IL1-R1-deficient cell line did not cause an obvious inflammatory response. 159 These models provide a platform for understanding fundamental molecular mechanisms that are related to healthy joint tissue and further exploring potential targets of OA treatment. A particularly inspiring direction is the development of Cas9 as a therapeutic molecule for curing hereditary diseases. 160 , 161 Delivery tools for gene-editing molecules include adeno-associated viral vectors, lentiviral vectors, lipid nanoparticles, and other nonviral vectors. 162 Clinical trials aimed at evaluating the ability of CRISPR/Cas9 to safely correct genetic diseases such as sickle cell disease (NCT03745287), β-thalassemia (NCT03655678), and Leber congenital amaurosis type 10 (NCT03872479) have been initiated. We recently investigated the roles of NGF, IL-1β, and MMP13 in OA development by deleting these genes in joint tissues using the CRISPR–Cas9 technique 163 , 164 , 165 (Fig. 3c ). The key findings of this study were that joint tissue NGF deficiency could efficiently eliminate joint pain but not structural OA damage, and deletion of these three potential harmful OA genes for OA (NGF, IL-1β, and MMP13) had a strong chondroprotective effect, inhibiting pathological damage of joint tissues and alleviating joint pain caused by destabilization of medial meniscus (DMM) surgery. 166 The flexibility, programmability, and high efficiency of CRISPR/Cas9 technology have promoted genome editing in studies ranging from fundamental science to translational medicine. Perhaps CRISPR/Cas9 could be used to treat OA.
Traditional genomic editing relies on DSB repair pathways, which can cause various side effects in cells, such as large deletions and complex rearrangements around the target, the emergence and expansion of p53-inactivating mutations, and chromosomal truncations. 167 , 168 , 169 The risks associated with permanent DNA modifications raise serious safety concerns regarding the clinical applications of the CRISPR/Cas9 system. The emergence of the CRISPR/Cas13 family, which cuts a target RNA via intrinsic RNase activity, provides another promising method for gene therapy 145 , 170 (Fig. 3b ). Currently, type A, type B, type D, type X, and type Y Cas13 family members have been identified. 171 , 172 Cas13 targeting has some advantages compared to Cas9; it avoids inducing DNA damage to cells and is theoretically reversible, since it affects RNA. In 2020, Yang and colleagues used CasRx, which is derived from type D, to knockdown Pten , Pcsk9 , and lncLstr in mouse hepatocytes and successfully modulate complex metabolic networks. 173 The researchers also targeted vascular endothelial growth factor A (VEGFA), an angiogenic growth factor, and suppressed choroidal neovascularization (CNV) in a mouse model of age-related macular degeneration (AMD). 174
It has been reported that overactivation of the Indian hedgehog (Ihh) pathway in chondrocytes strengthens the severity of OA. 175 However, knockout of the Ihh gene is undesirable for OA treatment since it is lethal in animals. 176 In this context, Cas13-mediated Ihh knockdown will be a more desired choice through cartilage-specific delivery in adults. OA-related factors, including matrix metalloproteinases (MMPs), inflammatory cytokines, and growth factors, could be potential targets of the CRISPR/Cas13 system 163 , 164 (Fig. 3c ).
Single-cell RNA sequencing
Synovial tissues mainly contain two types of cells: synovial fibroblasts (SFs) and macrophages. During OA initiation, synovial cells interact with macrophages/leukocytes and release inflammatory cytokines, leading to OA development. Thus, to determine the key genes in SFs involved in OA occurrence, it is important to identify subpopulations of cells and associated genes in OA development. RNA sequencing (RNA-seq) and single-cell RNA sequencing (scRNA-seq) could be very useful to fulfill this task. Through these approaches, we may be able to identify key genes associated with OA initiation and progression.
scRNA-seq data of SFs in OA in the GEO database were analyzed. In these studies, the genes and pathways associated with OA development were analyzed and identified by bioinformatics methods. In one of these studies, scRNA-seq data of SFs in OA patients were subjected to bioinformatics analyses, and OA-related genes were identified as those encoding ECM proteins and immune and cell adhesion molecules. Fibronectin 1 was identified as a key protein with functional changes during OA development. 177
One comprehensive study was conducted using human OA cartilage at different stages to analyze the single-cell profiles of OA chondrocytes. This study shows a transition from proliferating chondrocytes to prehypertrophic and hypertrophic chondrocytes (HTCs) and identified a new subpopulation of cells among HTCs. The researchers also identified novel marker genes for cartilage progenitor cells and showed the association of these progenitor cells with fibrocartilage chondrocytes through bioinformatics analysis. 178 A recent scRNA-seq study demonstrated that two marker genes (Col6a3 and ACTG1) were highly expressed in synovial tissues and were involved in focal adhesion. These two genes were upregulated during OA development, suggesting their roles in OA progression. 179
Lineage tracing
Lineage tracing has been successfully used in skeletal development studies. Several mouse models, such as Osx-CreER mice, Col2-CreER mice and Nestin-CreER mice, have been generated and used in lineage tracing studies. These mice were bred with ROSA26R-LacZ reporter, ROSA tdTomato or ROSA mT/mG reporter mice. The differentiation and migration of mesenchymal progenitor cells could be monitored by examining reporter activity and the fates of stage-selective subsets of osteoblast lineage cells. These studies revealed that perichondrial precursors, Osx -expressing cells or Col2 -expressing cells (Fig. 4a–c ) could migrate and differentiate into trabecular osteoblasts, osteocytes, and stromal cells inside the developing bone, and some of these cells were also associated with invading blood vessels and pericytes. 180 These cells can maintain their progenitor cell phenotype into late postnatal life. 181 Based on lineage tracing studies, we also learned that resting zone chondrocytes in the growth plate could migrate into the bone marrow underneath the growth plate and dedifferentiate into mesenchymal progenitor cells. These cells could further differentiate into osteoblasts, which are involved in bone formation. 182 In addition, these dedifferentiated progenitor cells could also differentiate into adipocytes and regulate osteoclast formation. 183 , 184 Although lineage tracing techniques have been successfully used in skeletal development studies, we know very little about how mesenchymal cells differentiate into synovial cells and articular chondrocytes under normal physiological or osteoarthritic conditions.
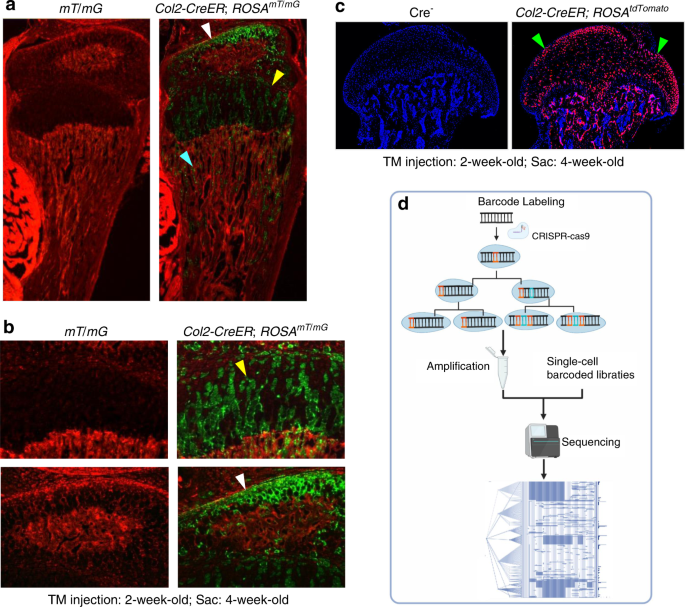
Lineage tracing technique. a – c Col2-expressing cells label a subpopulation of chondrocytes which could migrate to bone marrow underneath the growth plate and transdifferentiate into progenitor cells. a Col2-CreER mice were generated and bred with ROSA mT/mG reporter mice or ROSA tdTomato reporter mice. b Tamoxifen was intraperitoneally injected to the 2-week-old Col2-CreER; ROSA mT/mG mice or Col2-CreER; ROSA tdTomato mice for 5 consecutive days (1 mg per 10 g body weight) and mice were sacrificed at 4-week-old. c Col2-expressing cells were detected in articular chondrocytes, growth plate chondrocytes and cells located in bone marrow underneath the growth plate. d DNA barcoding technology was used for cell lineage tracing. Figure 4a, b 256 and Fig. 4c 257 were cited from our previous publications.
However, current lineage tracing approaches poorly reflect whole and complex organisms. In the past decade, the emergence and development of gene editing and DNA barcoding technologies have brought several advantages for cell lineage tracing 185 , 186 (Fig. 4d ). In 2016, McKenna and colleagues performed a combination of CRISPR/Cas9-based genome editing, DNA barcoding, and single-cell sequencing to read cell lineage information for the first time. 187 This technique has been called ‘dynamic lineage tracing’. 188 , 189 First, this approach marks individual cell lineages by inserting a compact DNA sequence into the genome. Then, the recombinase or CRISPR system is delivered or activated in cells to trigger barcode modification to increase the diversity of barcode sequences in cells during development. Finally, the barcode information is collected and analyzed to reconstruct a cell lineage tree by single-cell sequencing. 190
Cell death regulation
Cell death is a fundamental physiological process in multicellular organisms that maintains homeostasis during embryonic development and responds to harmful environmental stimuli to eliminate superfluous, damaged, senescent, and potentially harmful cells. 191 , 192 In contrast, programmed cell death is regulated by a complex and delicate genetic process and is beneficial for tissue homeostasis and immune responses. 193 , 194 For many years, apoptosis has long been considered the only form of regulated cell death, and necrosis was considered an unregulated cell death process. In the past two decades, the existence of new forms of cell death has been established. Several new forms of regulated cell death, such as pyroptosis, necroptosis, ferroptosis, parthanatos, mitochondrial permeability transition (MPT)-dependent necrosis, autophagy, and pyronecrosis, have been identified and characterized. 195 , 196 Recent findings have provided new insights into the mechanisms of regulated cell death and in vivo relevance to OA pathology and suggest that regulated cell death could have a significant impact on OA pathology (Fig. 5 ).
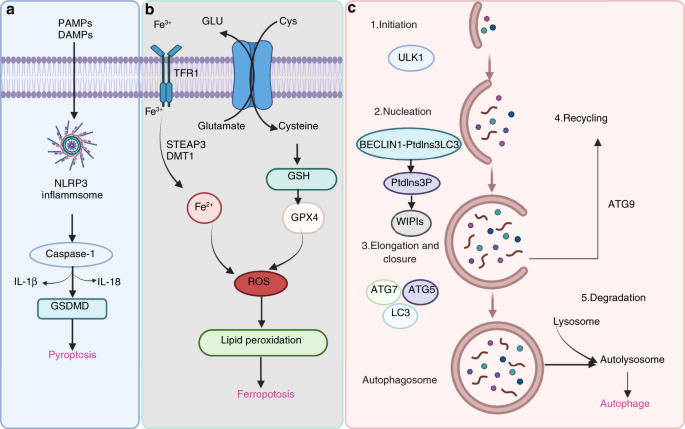
Pathways of pyroptosis, ferroptosis, and autophagy. a Many factors trigger assembly of the NLRP3 inflammasome, followed by activation of caspase-1 or caspase-11/4/5, which cleaves the gasdermin D (GSDMD) protein and pro-IL-1β and pro-IL-18, resulting in the release of IL-1β and IL-18. b During ferroptotic cell death, intracellular Glu is transported to the extracellular space, and extracellular Cys2 is transported into the cell, where it is then transformed into Cys for GSH synthesis. GPX4 reduces ROS accumulation. Excess iron is the basis for ferroptosis execution. Circulating iron binds with transferrin in the form of Fe 3+ and then enters the cells by TFR1. Fe 3+ is deoxidized to Fe 2+ by the iron oxide reductase STEAP3. Ultimately, Fe 2+ is released into a labile iron pool in the cytoplasm from the endosome via DMT1. c The canonical formation of autophagosomes involves the following steps: initiation, nucleation, elongation, closure, and recycling
Pyroptosis was identified by D’Souza et al. in 2001 and described as proinflammatory programmed cell death, which is different from apoptosis. 197 This process induces cell lysis and causes the massive release of cellular contents and proinflammatory factors, contributing to the development of many autoimmune and inflammatory diseases. Pyroptosis is triggered by the inflammasome-driven activation of caspase-1 or caspase-11/4/5, which cleaves the gasdermin D (GSDMD) protein, 198 , 199 , 200 separating its N-terminal pore-forming domain (GSDMD-NP) from the C-terminal repressor domain (GSDMD-CR). 201 , 202 GSDMD-NP binds to the inner acidic phospholipids of the plasma membrane and forms pores in the plasma membrane, resulting in the release of IL-1β and IL-18. These proinflammatory cytokines are then processed by caspase-1 and induce pyroptotic cell death (Fig. 5a ).
Excessive inflammation in chondrocytes plays a vital role in chondrocyte survival, leading to OA pathology. One study demonstrated that acid-sensitive ion channel 1a mediates chondrocyte pyroptosis in an adjuvant-induced arthritis model in rats. 203 Another study revealed that NLRP3 was activated in an experimental OA model, and the NLRP3 inhibitor CY-09 protected against OA development. 204 Our previous studies have demonstrated that loganin and morronside attenuate OA development by inhibiting chondrocyte pyroptosis. 26 , 205 As the main effector cells of synovial fibrosis in knee OA, fibroblast-like synoviocyte pyroptosis was reported to be mediated by NLRP1 and NLRP3 inflammasomes in knee OA, and an increase in HIF-1α may exacerbate synovial fibrosis by promoting fibroblast-like synoviocyte pyroptosis. 206 The molecular mechanisms underlying pyroptosis in OA need to be further investigated.
Ferroptosis
Ferroptosis is a novel form of programmed cell death that is driven by iron-dependent lipid peroxidation but is morphologically and biochemically different from other types of regulated cell death. 207 , 208 Dixon et al. first reported and named this nonapoptotic cell death, which is characterized by aberrant accumulation of lipid reactive oxygen species (ROS), in 2012. 209 Ferroptosis can be regulated by different metabolic pathways, including redox and iron homeostasis, mitochondrial activity and the metabolism of amino acids, lipids, and sugars. 210 , 211 GPX4 is the key regulator of ferroptosis. Ultimately, GPX4 inactivation results in iron-dependent intracellular accumulation of lethal levels of lipid hydroperoxides (Fig. 5b ).
Many pathophysiological processes are involved in the induction of ferroptosis, such as neurodegeneration, ischemia/reperfusion injury, and stroke. 212 , 213 In OA, inflammation and metabolic factors are two important contributors associated with OA cartilage loss and disease symptoms. 214 , 215 A recent report demonstrated that chondrocyte ferroptosis was involved in OA progression. 216 , 217 It has been demonstrated that the induction of chondrocyte ferroptosis increases MMP13 expression while decreasing collagen II expression. Intra-articular injection of ferrostatin-1, a ferroptosis inhibitor, prevented OA progression. Interestingly, several signaling pathways related to OA could regulate ferroptosis. Hippo-Yap signaling increases ferroptosis in cancer cells. 218 , 219 , 220 Energy stress-mediated AMPK signaling inhibits fatty acid synthesis and negatively regulates ferroptosis. 221 , 222 Hypoxia signaling promotes ferroptosis by activating hypoxia-inducible factors (HIFs) and increasing the production of ROS. 212 This evidence suggests that pharmacological modulation of ferroptosis may hold great potential for the treatment of OA.
Autophagy is a highly conserved process that results in lysosomal degradation of bulk cytoplasmic contents, abnormal macromolecule aggregates, and excess or damaged organelles, which is essential for cellular homeostasis. 223 , 224 In eukaryotic cells, autophagy often refers to macroautophagy, which is characterized by the generation of double-membraned vacuoles called autophagosomes, which sequester cytoplasmic components before delivering them to the lysosome for degradation. The canonical formation of autophagosomes involves the following steps: initiation, nucleation, elongation and closure and recycling (Fig. 5c ). Each step depends on a unique molecular mechanism. The important components of the autophagy signaling pathway include the ULK1 complex, the BECLIN1-PtdIns3KC3-ATG14L complex, WIPIs, ATG12-ATG5, LC3-PE conjugation systems, and ATG9. 223 Activating mTOR suppresses autophagy and Akt and AMPK signaling, and inhibiting mTOR promotes AMPK and p53 signaling. 225 , 226 , 227 In addition, hypoxia, reactive oxygen species and nutrient and energy deprivation can generally activate autophagy.
It is well known that OA is an aging-related disease associated with the accumulation of damaged macromolecules, which leads to chondrocyte dysfunction and death. Previous studies have reported that reduced expression of ULK1, Beclin1, and LC3 was observed in human OA and aging-related and surgically induced OA in mice, indicating a deficiency in autophagy regulation in OA chondrocytes. 228 Recent studies have demonstrated that autophagy activation protects against mitochondrial dysfunction in human chondrocytes and that loss of function of mTOR in cartilage tissue protects mice from developing OA by upregulating autophagy. 229 , 230 Thus, the use of therapeutics targeting the autophagy signaling pathway is a potential strategy for OA treatment. Certain autophagy-enhancing drugs have been shown to attenuate OA cartilage degeneration. Local intraarticular injection of rapamycin reduces mTOR expression and delays articular cartilage degeneration in a DMM-induced murine model of OA. 42 , 231 Currently, a phase III clinical trial (NCT02905799) is being conducted to determine the efficacy of resveratrol for OA treatment.
Synovial lymphatics in osteoarthritis
Currently, there is no effective therapy for OA. The identification of new pathways and mechanisms responsible for the initiation and progression of OA will advance the development of new therapies for OA. Cartilage-derived catabolic factors accumulate in the synovium of OA joints, but how these catabolic factors are cleared is currently unknown.
The synovial lymphatic system (SLS)
Lymphatic vessels (LVs) are composed of capillary and collecting vessels. 232 Capillary LVs have a thin layer of lymphatic endothelial cells (LECs) that express LV endothelial hyaluronan receptor 1 and podoplanin, a transmembrane glycoprotein. 233 Collecting LVs are covered with lymphatic muscle cells that have phenotypic features of both striated and vascular smooth muscle cells. 234 , 235 However, a recent lineage tracing study revealed that popliteal lymphatic muscle progenitor cells are distinct from skeletal (Pax7 + and MyoD + ) and vascular muscle progenitors (Prrx1 + and NG2 + ) during development and after postnatal Day 10 and are derived from a previously unknown Pax7 - /MyoD - /Prrx1 + /NG2 − muscle progenitor. 236 Lymph cells are propelled by alternating contraction and relaxation of lymphatic muscle cells to draining lymph nodes (DLNs) and eventually to the venous circulation. 237 , 238
Using a combination of near-infrared and indocyanine green lymphatic imaging 239 , 240 , 241 , 242 , 243 , 244 to monitor LV contraction and clearance from joints to DLNs and immunofluorescence staining/whole slide imaging to identify and qualify LVs in mouse joints, 245 , 246 we reported that LVs are present in the synovium and surrounding soft tissues in the knee joint, including the joint capsule, fat pads, ligaments, and muscles. Knees drain to iliac LNs (ILNs), while ankles (footpad) drain to popliteal LNs (PLNs) via collecting LVs. 241 , 245 , 247 These LVs are therefore named the synovial lymphatic system (SLS), which consists of initial LVs in the synovium and the surrounding soft tissues, DLNs, and collecting LVs (large vessels whose contractions move lymph from initial LVs to DLNs). 248
The SLS in arthritis
The role of the SLS in murine and human rheumatoid arthritis (RA), which is an autoimmune disease, has been actively studied in the past 15 years, and the results reveal its importance in RA progression and treatment (for details please see the review). 248 This conclusion is based on several reports. (a) In RA mouse models, including collagen-induced arthritis mice, KRN transgenic mice and TNF transgenic mice, SLS draining correlates with the progression of joint damage and is improved by drugs that reduce RA pathology. (b) Stimulation of lymphangiogenesis by vascular endothelial growth factor C (VEGF-C) and its receptor (VEGFR3) attenuates RA joint damage, and inhibiting lymphangiogenesis with a VEGF inhibitor accelerates RA joint damage. VEGF-C is considered a lymphatic-specific growth factor because VEGFR3 is mainly expressed by LECs. (c) More recently, altered LV anatomy and markedly decreased lymph clearance were observed in the affected hands of individuals with active RA, 249 providing strong evidence for the involvement of the SLS in human RA. OA patients suffer from similar joint stiffness and pain as RA individuals, but these types of arthritis are very different in origin. OA is associated with long-term mechanical wear and tear on the cartilage during natural aging, while RA is an autoimmune disease. The involvement of the SLS in human and murine RA has been well studied; however, only a few reports have focused on the SLS in OA.
SLS studies in OA
Early case studies reported that a radiolabeled tracer was cleared to DLNs after being injected into a normal joint, and it accumulated in the synovial space following injection into an OA joint of the same individual. 250 These findings suggested that the injected tracer was removed from the synovial space via LVs in normal joints and that this process was impaired in OA joints.
A more systemic clinical study was performed by immunostaining 60 knee samples taken from severe knee OA patients during total knee replacement surgery. Sixty postmortem control knees were stained with podoplanin, a commonly used marker for LECs, and LV density and LEC fractional area were quantified. Similar results were obtained as those found in a mouse study. 245 LVs were detected in synovial tissue but not in subchondral bone. OA samples exhibited lower LV density and fewer LEC fractional areas than nonarthritic controls. In individuals with OA, low LV density and LEC fractional areas were associated with clinically detectable effusion but not with radiological or histological severity of OA tissue damage. These findings support a pathogenic role of synovial drainage via LVs in effusion as a clinical sign of synovitis in OA. 251
SLS studies in OA mouse models
To investigate whether the SLS contributes to OA pathology and identify the potential mechanisms involved, we examined lymphatic drainage in WT mice with posttraumatic OA (PTOA) and found that lymphatic drainage was decreased in PTOA joints. 245 VEGFR3-neutralizing antibody administration further reduced synovial lymphatic drainage and accelerated joint tissue damage. Synovial LECs that were isolated from PTOA joints based on podoplanin expression had increased expression of inflammatory factors. Interestingly, more macrophages, mainly M1 macrophages, were located adjacent to podoplanin + LVs, and these M1 macrophages stimulated inflammatory gene expression in LECs in cocultures. Intra-articular injection of bortezomib increased lymphatic drainage and decreased the number of M1 macrophages in synovial tissue, resulting in decreased cartilage loss. Thus, local joint delivery of bortezomib or other anti-inflammatory drugs may restore synovial lymphatic function in individuals with posttraumatic knee OA. 25
OA develops primarily as a result of aging. Interestingly, lymphatic dysfunction in aging has been recognized recently. In 2018, Mesquita et al. reported that in the brain, meningeal LVs remove cerebrospinal fluid macromolecules. Disruption of meningeal LVs in mouse models of Alzheimer’s disease promoted amyloid deposition and exacerbated parenchymal amyloid accumulation. 252 Treatment of aged mice or mice with Alzheimer’s disease with VEGF-C enhanced meningeal lymphatic drainage. This study also showed decreased VEGFR3 signaling in meningeal LECs isolated from aged mice. Thus, it is likely that VEGF-C/VEGFR3 downregulation represents a new underlying mechanism for age-related lymphatic dysfunction.
Arthritogenic matrix metalloproteinase (MMP13) is cleared from arthritic joints via the SLS
The SLS drains large molecules to DLNs. MMP13 is a critical catabolic enzyme in both RA and OA arthritic joints that destroys cartilage. Despite some beneficial effects on arthritic models, 253 MMP13 inhibitors have shown no greater advantages than placebo in OA clinical trials, and notable musculoskeletal toxicity, such as arthralgia and edema, was observed. 254 To examine whether the MMP13 protein can be cleared from arthritic joints to DLNs via the SLS, we examined MMP13 protein and gene expression in the synovium and corresponding DLNs by immunohistochemistry (IHC) and RT–qPCR in PTOA or TNF transgenic (TNF-Tg) mice. MMP13 protein and mRNA expression was significantly higher in the synovium of PTOA and RA mice than in controls. MMP13 protein levels in the DLNs of arthritic mice were also markedly upregulated compared to those of control mice, but no differences in mRNA levels were detected (Fig. 6 ). The IHC results showed that MMP13 was expressed on only articular chondrocytes in mice with PTOA and the synovium in TNF-Tg mice (Fig. 6 a, d). Consistently, the DLNs in these two established mouse models exhibited robust immunoreactivity, while no MMP13 expression was detected in the DLNs of control mice without arthritis (Fig. 6a–e ). Interestingly, we only detected the expression of Mmp13 mRNA in the knee synovium but not in DLNs (Fig. 6 c, f). Given the high MMP13 protein expression in DLNs without de novo synthesis, these data demonstrate that matrix metalloproteinases expressed in the DLNs came from the afferent arthritic knee and was translocated via SLS clearance. Furthermore, the SLS plays an important role in the efficient clearance of catabolic large molecules that lead to cartilage breakup, which is critical for protecting against arthritic damage. Thus, improving lymphatic clearance is a viable strategy for arthritis treatment.
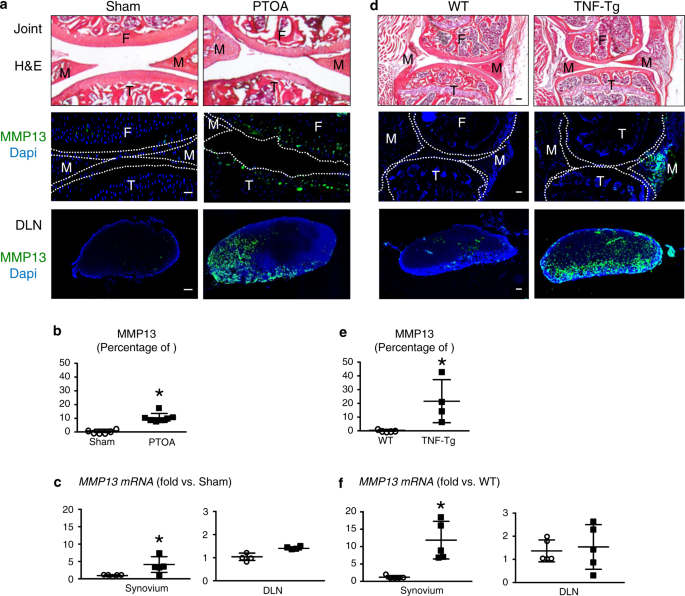
Arthritogenic MMP13 is removed from osteoarthritic and inflamed joints via the synovial lymphatic system. C57BL/6J mice received sham or DMM surgery ( a – c ), and TNF-Tg and WT control mice ( d – f ) were used. Knees and DLNs were harvested from operated mice 6 weeks post-surgery ( a – c ) and from 6-month-old WT and TNF-Tg mice ( d – f ). a , d Frozen sections from the knees were H&E stained for light microscopy, and representative 10x images are shown (scale bar = 100 µm). F: femur, T: tibia, M: meniscus. Parallel knee sections (dotted lines indicate the joint space) and DLN sections were immunostained with green fluorescent labeled antibodies against MMP13, counterstained with DAPI, and representative dark field images obtained at 10x are shown. Note that only articular chondrocytes in PTOA knees, synovium in TNF-Tg knees, and their DLNs, have robust MMP13 immuno-reactivity. b , e VisioPharm histomorphometry was performed to quantify the percentage of MMP13 + area of the DLN immunostained sections, and data are presented for each DLN +/− SD (* P < 0.05 vs. non-arthritic control with paired t -test). c , f Mmp13 mRNA levels in knees were assessed via qPCR. The data are presented for each tissue ± SD (* P < 0.05 vs. non-arthritic control with paired t -test). The content presented in Fig. 6 was derived from a PhD dissertation and was provided by Dr. Xi Lin, with her permission, a postdoctoral fellow in University of Rochester Medical Center 258
Most SLS studies are focused on the association between the SLS and arthritis during disease progression and the response to treatment. For example, SLS dysfunction becomes more severe with arthritis progression, and drugs that attenuate joint damage are often associated with the restoration of SLS function. In these studies, the specific involvement of LVs in arthritis is evidenced by LEC-specific manipulation, including the use of VEGF-C- and VEGFR3-neutralizing antibodies or signaling inhibitors. Since the VEGF-C/VEGFR3 signaling pathway affects processes than those of LECs, such as macrophage activation, 255 using a genetically modified mouse model with LEC-specific manipulation is needed to provide more direct evidence of the causal role of the SLS in arthritis pathogenesis. Currently, a major mechanism for SLS dysfunction in RA and OA is associated with inflammation. In synovial tissue, inflammatory factors and cells such as macrophages can affect LV cell function. Furthermore, LV cells, including LECs and lymphatic muscle cells, can be inflamed, resulting in functional changes. It will be very interesting to identify molecular signatures of LECs and lymphatic muscle cells in an arthritic microenvironment and discover new pathological genes/pathways that are intrinsically expressed by LV cells.
Conclusions
Over the past decades, our understanding of OA pathogenesis has expanded from OA being a ‘wear and tear’ disease to whole joint pathology featuring synovitis, cartilage damage, subchondral bone remodeling, and osteophyte formation. OA pathology involves a variety of factors, such as mechanical loading, aging, inflammation and metabolic changes, and the activation of different signaling pathways, such as Wnt/β-catenin, Ihh, TGF-β, EGFR, HIF, NF-κB, and Notch. New approaches and novel techniques have been explored and developed in OA research from different aspects. Epigenetic regulation, such as DNA methylation, histone modification, and miRNA regulation, provides new insights into the pathogenesis of OA at the transcriptional and/or posttranscriptional level. A variety of cell death types have been observed in OA development and have significant impacts on OA pathology. The synovial lymphatic system plays an important role in the clearance of cartilage-derived catabolic factors in the synovium of OA joints, which opens up novel research directions. Novel techniques, such as CRISPR/Cas9 genome editing, single-cell RNA sequencing, and lineage tracing, have been developed and used in OA studies, and these techniques greatly facilitates the discovery of new methods and drug candidates for OA treatment. Despite the significant progress in our understanding of OA pathogenesis, the etiology and pathological mechanisms of OA are not yet fully understood. It is conceivable that the application of novel techniques could accelerate our understanding of OA pathogenesis. In the future, it will be crucial to explore the molecular mechanisms underlying OA-associated pain and articular pathology as well as the relationships between them, which would be helpful in developing more specific and effective therapeutic interventions for OA.
Chen, D. Osteoarthritis: a complicated joint disease requiring extensive studies with multiple approaches. J. Orthop. Translat. 32 , 130 (2022).
Article PubMed PubMed Central Google Scholar
Chen, D. et al. Osteoarthritis: toward a comprehensive understanding of pathological mechanism. Bone Res. 5 , 16044 (2017).
Article CAS PubMed PubMed Central Google Scholar
Li, Y., Xie, W., Xiao, W. & Dou, D. Progress in osteoarthritis research by the National Natural Science Foundation of China. Bone Res. 10 , 41 (2022).
Sun, A. R. et al. Cartilage tissue engineering for obesity-induced osteoarthritis: physiology, challenges, and future prospects. J. Orthop. Translat. 26 , 3–15 (2021).
Article PubMed Google Scholar
Wu, X. H., Lai, Y. M., Cao, H. L. & Xiao, G. Z. Kindlin-2 preserves integrity of the articular cartilage to protect against osteoarthritis. J. Bone Min. Res. 37 , 45–46 (2022).
Google Scholar
Wen, C. & Xiao, G. Advances in osteoarthritis research in 2021 and beyond. J. Orthop. Translat. 32 , A1–A2 (2022).
GBD 2017 Disease and Injury Incidence and Prevalence Collaborators. Global, regional, and national incidence, prevalence, and years lived with disability for 354 diseases and injuries for 195 countries and territories, 1990-2017: a systematic analysis for the Global Burden of Disease Study 2017. Lancet 392 , 1789–1858 (2018).
Article Google Scholar
Li, J., Wang, Y., Chen, D. & Liu-Bryan, R. Oral administration of berberine limits post-traumatic osteoarthritis development and associated pain via AMP-activated protein kinase (AMPK) in mice. Osteoarthr. Cartil. 30 , 160–171 (2022).
Article CAS Google Scholar
Li, J. et al. Metformin limits osteoarthritis development and progression through activation of AMPK signalling. Ann. Rheum. Dis. 79 , 635–645 (2020).
Article CAS PubMed Google Scholar
Lu, K. et al. Molecular signaling in temporomandibular joint osteoarthritis. J. Orthop. Translat. 32 , 21–27 (2022).
Katz, J. N., Arant, K. R. & Loeser, R. F. Diagnosis and treatment of hip and knee osteoarthritis: a review. JAMA 325 , 568–578 (2021).
Ni, R., Guo, X. E., Yan, C. & Wen, C. Hemodynamic stress shapes subchondral bone in osteoarthritis: An emerging hypothesis. J. Orthop. Translat. 32 , 85–90 (2022).
McHugh, J. Osteoarthritis risk factors differ between sexes. Nat. Rev. Rheumatol. 17 , 312 (2021).
PubMed PubMed Central Google Scholar
Huang, W., Ong, T. Y., Fu, S. C. & Yung, S. H. Prevalence of patellofemoral joint osteoarthritis after anterior cruciate ligament injury and associated risk factors: a systematic review. J. Orthop. Translat. 22 , 14–25 (2020).
Fang, L. et al. Defining disease progression in Chinese mainland people: association between bone mineral density and knee osteoarthritis. J. Orthop. Translat. 26 , 39–44 (2021).
Hunter, D. J. & Bierma-Zeinstra, S. Osteoarthritis. Lancet 393 , 1745–1759 (2019).
Chen, L. et al. Pathogenesis and clinical management of obesity-related knee osteoarthritis: Impact of mechanical loading. J. Orthop. Translat. 24 , 66–75 (2020).
Meurot, C. et al. Targeting the GLP-1/GLP-1R axis to treat osteoarthritis: a new opportunity? J. Orthop. Translat. 32 , 121–129 (2022).
Chen, D., Kim, D. J., Shen, J., Zou, Z. & O’Keefe, R. J. Runx2 plays a central role in Osteoarthritis development. J. Orthop. Translat. 23 , 132–139 (2020).
Yu, H., Huang, T., Lu, W. W., Tong, L. & Chen, D. Osteoarthritis Pain. Int. J. Mol. Sci. 23 , 4642 (2022).
Li, J., Ma, K., Yi, D., Oh, C. D. & Chen, D. Nociceptive behavioural assessments in mouse models of temporomandibular joint disorders. Int. J. Oral Sci. 12 , 26 (2020).
Zhou, F. et al. Associations of osteoclastogenesis and nerve growth in subchondral bone marrow lesions with clinical symptoms in knee osteoarthritis. J. Orthop. Translat. 32 , 69–76 (2022).
Yang, J. et al. Targeting cell death: pyroptosis, ferroptosis, apoptosis and necroptosis in osteoarthritis. Front. Cell Dev. Biol. 9 , 789948 (2021).
Cao, M., Ong, M. T. Y., Yung, P. S. H., Tuan, R. S. & Jiang, Y. Role of synovial lymphatic function in osteoarthritis. Osteoarthr. Cartil. (2022).
Wang, W. et al. Attenuated joint tissue damage associated with improved synovial lymphatic function following treatment with bortezomib in a mouse model of experimental posttraumatic osteoarthritis. Arthritis Rheumatol. 71 , 244–257 (2019).
Yu, H. et al. Morroniside attenuates apoptosis and pyroptosis of chondrocytes and ameliorates osteoarthritic development by inhibiting NF-κB signaling. J. Ethnopharmacol. 266 , 113447 (2021).
Simon, T. C. & Jeffries, M. A. The epigenomic landscape in osteoarthritis. Curr. Rheumatol. Rep. 19 , 30 (2017).
Article PubMed PubMed Central CAS Google Scholar
Zhang, M. & Wang, J. Epigenetics and osteoarthritis. Genes Dis. 2 , 69–75 (2015).
Roach, H. I. & Aigner, T. DNA methylation in osteoarthritic chondrocytes: a new molecular target. Osteoarthr. Cartil. 15 , 128–137 (2007).
Miranda-Duarte, A. DNA methylation in osteoarthritis: current status and therapeutic implications. Open Rheumatol. J. 12 , 37–49 (2018).
Morris, M. J. & Monteggia, L. M. Role of DNA methylation and the DNA methyltransferases in learning and memory. Dialogues Clin. Neurosci. 16 , 359–371 (2014).
Li, E., Bestor, T. H. & Jaenisch, R. Targeted mutation of the DNA methyltransferase gene results in embryonic lethality. Cell 69 , 915–926 (1992).
Okano, M., Bell, D. W., Haber, D. A. & Li, E. DNA methyltransferases Dnmt3a and Dnmt3b are essential for de novo methylation and mammalian development. Cell 99 , 247–257 (1999).
Jeffries, M. A. et al. Genome-wide DNA methylation study identifies significant epigenomic changes in osteoarthritic cartilage. Arthritis Rheumatol. 66 , 2804–2815 (2014).
Raman, S., FitzGerald, U. & Murphy, J. M. Interplay of inflammatory mediators with epigenetics and cartilage modifications in osteoarthritis. Front. Bioeng. Biotechnol. 6 , 22 (2018).
Chen, D., Shen, J. & Hui, T. Epigenetic and microRNA regulation during osteoarthritis development. F1000Res. 4 , F1000 Faculty Rev−1092 (2015).
Cheung, K. S., Hashimoto, K., Yamada, N. & Roach, H. I. Expression of ADAMTS-4 by chondrocytes in the surface zone of human osteoarthritic cartilage is regulated by epigenetic DNA de-methylation. Rheumatol. Int. 29 , 525–534 (2009).
Hashimoto, K., Oreffo, R. O., Gibson, M. B., Goldring, M. B. & Roach, H. I. DNA demethylation at specific CpG sites in the IL1B promoter in response to inflammatory cytokines in human articular chondrocytes. Arthritis Rheumatol. 60 , 3303–3313 (2009).
Roach, H. I. et al. Association between the abnormal expression of matrix-degrading enzymes by human osteoarthritic chondrocytes and demethylation of specific CpG sites in the promoter regions. Arthritis Rheumtol. 52 , 3110–3124 (2005).
Shen, J., Abu-Amer, Y., O’Keefe, R. J. & McAlinden, A. Inflammation and epigenetic regulation in osteoarthritis. Connect. Tissue Res. 58 , 49–63 (2017).
Zimmermann, P. et al. Correlation of COL10A1 induction during chondrogenesis of mesenchymal stem cells with demethylation of two CpG sites in the COL10A1 promoter. Arthritis Rheumatol. 58 , 2743–2753 (2008).
Tan, L. & Shi, Y. G. Tet family proteins and 5-hydroxymethylcytosine in development and disease. Development 139 , 1895–1902 (2012).
Andrés, M. D. et al. Loss of methylation in CpG sites in the NF-κB enhancer elements of inducible nitric oxide synthase is responsible for gene induction in human articular chondrocytes. Arthritis Rheumtol. 65 , 732–742 (2014).
Lin, X. et al. Genome-wide analysis of aberrant methylation of enhancer DNA in human osteoarthritis. BMC Med. Genomics 13 , 1 (2020).
Whalen, S., Truty, R. M. & Pollard, K. S. Enhancer-promoter interactions are encoded by complex genomic signatures on looping chromatin. Nat. Genet. 48 , 488–496 (2016).
Zhu, X. et al. PPARgamma preservation via promoter demethylation alleviates osteoarthritis in mice. Ann. Rheum. Dis. 78 , 1420–1429 (2019).
Deng, Z. et al. Losartan protects against osteoarthritis by repressing the TGF-beta1 signaling pathway via upregulation of PPARgamma. J. Orthop. Translat. 29 , 30–41 (2021).
Shen, J. et al. DNA methyltransferase 3b regulates articular cartilage homeostasis by altering metabolism. JCI Insight. 2 , e93612 (2017).
Zhou, Y. et al. Epigenetic and therapeutic implications of dnmt3b in temporomandibular joint osteoarthritis. Am. J. Transl. Res. 11 , 1736–1747 (2019).
CAS PubMed PubMed Central Google Scholar
Blanco, F. J. & Rego-Perez, I. Editorial: Is it time for epigenetics in osteoarthritis? Arthritis Rheumatol. 66 , 2324–2327 (2014).
Kim, H., Kang, D., Cho, Y. & Kim, J. H. Epigenetic regulation of chondrocyte catabolism and anabolism in osteoarthritis. Mol. Cells 38 , 677–684 (2015).
Bradley, E. W., Carpio, L. R., van Wijnen, A. J., McGee-Lawrence, M. E. & Westendorf, J. J. Histone deacetylases in bone development and skeletal disorders. Physiol. Rev. 95 , 1359–1381 (2015).
Cao, K. et al. Decreased histone deacetylase 4 is associated with human osteoarthritis cartilage degeneration by releasing histone deacetylase 4 inhibition of runt-related transcription factor-2 and increasing osteoarthritis-related genes: a novel mechanism of human osteoarthritis cartilage degeneration. Arthritis Res. Ther. 16 , 491 (2014).
El Mansouri, F. et al. Lysine-specific demethylase 1-mediated demethylation of histone H3 lysine 9 contributes to interleukin 1β-induced microsomal prostaglandin E synthase 1 expression in human osteoarthritic chondrocytes. Arthritis Res. Ther. 16 , R113 (2014).
El Mansouri, F. E. et al. Contribution of H3K4 methylation by SET-1A to interleukin-1-induced cyclooxygenase 2 and inducible nitric oxide synthase expression in human osteoarthritis chondrocytes. Arthritis Rheumatol. 63 , 168–179 (2011).
Higashiyama, R. et al. Correlation between MMP-13 and HDAC7 expression in human knee osteoarthritis. Mod. Rheumatol. 20 , 11–17 (2010).
Kim, K. I., Park, Y. S. & Im, G. I. Changes in the epigenetic status of the SOX-9 promoter in human osteoarthritic cartilage. J. Bone Miner. Res. 28 , 1050–1060 (2013).
Hong, S., Derfoul, A., Pereira-Mouries, L. & Hall, D. J. A novel domain in histone deacetylase 1 and 2 mediates repression of cartilage-specific genes in human chondrocytes. FASEB J. 23 , 3539–3552 (2009).
Huber, L. C. et al. Histone deacetylase/acetylase activity in total synovial tissue derived from rheumatoid arthritis and osteoarthritis patients. Arthritis Rheumatol. 56 , 1087–1093 (2007).
Huh, Y. H., Ryu, J. H. & Chun, J. S. Regulation of type II collagen expression by histone deacetylase in articular chondrocytes. J. Biol. Chem. 282 , 17123–17131 (2007).
Lu, J., Sun, Y., Ge, Q., Teng, H. & Jiang, Q. Histone deacetylase 4 alters cartilage homeostasis in human osteoarthritis. BMC Musculoskelet. Disord. 15 , 438 (2014).
Chang, L. H., Chen, C. H., Wu, S. C., Chang, J. K. & Ho, M. L. Cyclooxygenase-2 regulates PTHrP transcription in human articular chondrocytes and is involved in the pathophysiology of osteoarthritis in rats. J. Orthop. Translat. 30 , 16–30 (2021).
Im, G. I. & Choi, Y. J. Epigenetics in osteoarthritis and its implication for future therapeutics. Expert Opin. Biol. Ther. 13 , 713–721 (2013).
Cai, D., Yin, S., Yang, J., Jiang, Q. & Cao, W. Histone deacetylase inhibition activates Nrf2 and protects against osteoarthritis. Arthritis Res. Ther. 17 , 269 (2015).
Culley, K. L. et al. Class I histone deacetylase inhibition modulates metalloproteinase expression and blocks cytokine-induced cartilage degradation. Arthritis Rheumatol. 65 , 1822–1830 (2013).
Khan, N. M. & Haqqi, T. M. Epigenetics in osteoarthritis: potential of HDAC inhibitors as therapeutics. Pharmacol. Res. 128 , 73–79 (2018).
Article PubMed CAS Google Scholar
Makki, M. S. & Haqqi, T. M. Histone deacetylase inhibitor vorinostat (SAHA) suppresses IL-1beta-induced matrix metallopeptidase-13 expression by inhibiting IL-6 in osteoarthritis chondrocyte. Am. J. Pathol. 186 , 2701–2708 (2016).
Wang, X., Song, Y., Jacobi, J. L. & Tuan, R. S. Inhibition of histone deacetylases antagonized FGF2 and IL-1beta effects on MMP expression in human articular chondrocytes. Growth Factors 27 , 40–49 (2009).
Zhong, H. M., Ding, Q. H., Chen, W. P. & Luo, R. B. Vorinostat, a HDAC inhibitor, showed anti-osteoarthritic activities through inhibition of iNOS and MMP expression, p38 and ERK phosphorylation and blocking NF-kappaB nuclear translocation. Int. Immunopharmacol. 17 , 329–335 (2013).
Liu, T. F., Yoza, B. K., El Gazzar, M., Vachharajani, V. T. & McCall, C. E. NAD+-dependent SIRT1 deacetylase participates in epigenetic reprogramming during endotoxin tolerance. J. Biol. Chem. 286 , 9856–9864 (2011).
Matsuzaki, T. et al. Disruption of Sirt1 in chondrocytes causes accelerated progression of osteoarthritis under mechanical stress and during ageing in mice. Ann. Rheum. Dis. 73 , 1397–1404 (2014).
Moon, M. H. et al. SIRT1, a class III histone deacetylase, regulates TNF-alpha-induced inflammation in human chondrocytes. Osteoarthr. Cartil. 21 , 470–480 (2013).
Takayama, K. et al. SIRT1 regulation of apoptosis of human chondrocytes. Arthritis Rheumatol. 60 , 2731–2740 (2009).
Matsushita, T. et al. The overexpression of SIRT1 inhibited osteoarthritic gene expression changes induced by interleukin-1beta in human chondrocytes. J. Orthop. Res. 31 , 531–537 (2013).
Deng, Z. et al. The role of sirtuin 1 and its activator, resveratrol in osteoarthritis. Biosci. Rep. 39 , BSR20190189 (2019).
Kim, H. J., Braun, H. J. & Dragoo, J. L. The effect of resveratrol on normal and osteoarthritic chondrocyte metabolism. Bone Jt. Res. 3 , 51–59 (2014).
Lei, M. et al. Resveratrol inhibits interleukin 1beta-mediated inducible nitric oxide synthase expression in articular chondrocytes by activating SIRT1 and thereby suppressing nuclear factor-kappaB activity. Eur. J. Pharmacol. 674 , 73–79 (2012).
Lim, H. D. et al. Cytoprotective and anti-inflammatory effects of melatonin in hydrogen peroxide-stimulated CHON-001 human chondrocyte cell line and rabbit model of osteoarthritis via the SIRT1 pathway. J. Pineal Res. 53 , 225–237 (2012).
Marouf, B. H., Hussain, S. A., Ali, Z. S. & Ahmmad, R. S. Resveratrol supplementation reduces pain and inflammation in knee osteoarthritis patients treated with meloxicam: a randomized placebo-controlled study. J. Med. Food (2018).
Nishida, K. et al. Intraperitoneal injection of the SIRT1 activator SRT1720 attenuates the progression of experimental osteoarthritis in mice. Bone Jt. Res. 7 , 252–262 (2018).
Krol, J., Loedige, I. & Filipowicz, W. The widespread regulation of microRNA biogenesis, function and decay. Nat. Rev. Genet. 11 , 597–610 (2010).
Du, X. F. et al. Role of the miR-133a-5p/FBXO6 axis in the regulation of intervertebral disc degeneration. J. Orthop. Translat. 29 , 123–133 (2021).
MacFarlane, L. A. & Murphy, P. R. MicroRNA: biogenesis, function and role in cancer. Curr. Genomics 11 , 537–561 (2010).
Friedman, R. C., Farh, K. H., Burge, C. B. & Bartel, D. P. Most mammalian mRNAs are conserved targets of microRNAs. Genome Res. 19 , 92–105 (2008).
Benz, F., Roy, S., Trautwein, C., Roderburg, C. & Luedde, T. Circulating MicroRNAs as Biomarkers for Sepsis. Int. J. Mol. Sci. 17 , 78 (2016).
Article PubMed Central CAS Google Scholar
Coutinho de Almeida, R. et al. RNA sequencing data integration reveals an miRNA interactome of osteoarthritis cartilage. Ann. Rheum. Dis. 78 , 270–277 (2019).
Yoshida, C. A. et al. Runx2 and Runx3 are essential for chondrocyte maturation, and Runx2 regulates limb growth through induction of Indian hedgehog. Gene Dev. 18 , 952–963 (2004).
Kamekura, S. et al. Contribution of runt-related transcription factor 2 to the pathogenesis of osteoarthritis in mice after induction of knee joint instability. Arthritis Rheumatol. 54 , 2462–2470 (2010).
Liao, L. et al. Deletion of Runx2 in articular chondrocytes decelerates the progression of DMM-induced osteoarthritis in adult mice. Sci. Rep. 7 , 2371 (2017).
Huang, J., Zhao, L., Xing, L. & Chen, D. MicroRNA-204 regulates Runx2 protein expression and mesenchymal progenitor cell differentiation. Stem Cells 28 , 357–364 (2010).
Huang, J. et al. The microRNAs miR-204 and miR-211 maintain joint homeostasis and protect against osteoarthritis progression. Nat. Commun. 10 , 2876 (2019).
Zhu, S. et al. Coupling factors and exosomal packaging microRNAs involved in the regulation of bone remodelling. Biol. Rev. Camb. Philos. Soc. 93 , 469–480 (2018).
Nakamura, A. et al. microRNA-181a-5p antisense oligonucleotides attenuate osteoarthritis in facet and knee joints. Ann. Rheum. Dis. 78 , 111–121 (2019).
Wu, D. et al. Brain endothelial miR-146a negatively modulates T-cell adhesion through repressing multiple targets to inhibit NF-κB activation. J. Cereb. Blood Flow. Metab. 35 , 412–423 (2015).
Zhong, G. et al. miRNA-335-5p relieves chondrocyte inflammation by activating autophagy in osteoarthritis. Life Sci. 226 , 164–172 (2019).
Ding, Y., Wang, L., Zhao, Q., Wu, Z. & Kong, L. MicroRNA93 inhibits chondrocyte apoptosis and inflammation in osteoarthritis by targeting the TLR4/NF-κB signaling pathway. Int. J. Mol. Med. 43 , 779–790 (2019).
CAS PubMed Google Scholar
Bjurstrom, M. F. et al. Central nervous system monoaminergic activity in hip osteoarthritis patients with disabling pain: associations with pain severity and central sensitization. Pain. Rep. 7 , e988 (2022).
Gungor Demir, U., Demir, A. N. & Toraman, N. F. Neuropathic pain in knee osteoarthritis. Adv. Rheumatol. 61 , 67 (2021).
Graven-Nielsen, T., Wodehouse, T., Langford, R. M., Arendt-Nielsen, L. & Kidd, B. L. Normalization of widespread hyperesthesia and facilitated spatial summation of deep-tissue pain in knee osteoarthritis patients after knee replacement. Arthritis Rheumatol. 64 , 2907–2916 (2012).
Manni, L. et al. Nerve growth factor: basic studies and possible therapeutic applications. Growth Factors 31 , 115–122 (2013).
Hirose, M., Kuroda, Y. & Murata, E. NGF/TrkA signaling as a therapeutic target for pain. Pain Pract. 16 , 175–182 (2016).
Norman, B. H. & McDermott, J. S. Targeting the nerve growth factor (NGF) pathway in drug discovery. Potential applications to new therapies for chronic pain. J. Med. Chem. 60 , 66–88 (2017).
Takatori, M., Kuroda, Y. & Hirose, M. Local anesthetics suppress nerve growth factor-mediated neurite outgrowth by inhibition of tyrosine kinase activity of TrkA. Anesth. Analg. 102 , 462–467 (2006).
Dakin, P. et al. The efficacy, tolerability, and joint safety of fasinumab in osteoarthritis pain: a phase IIb/III double-blind, placebo-controlled, randomized clinical trial. Arthritis Rheumatol. 71 , 1824–1834 (2019).
Berenbaum, F. et al. Subcutaneous tanezumab for osteoarthritis of the hip or knee: efficacy and safety results from a 24-week randomised phase III study with a 24-week follow-up period. Ann. Rheum. Dis. 79 , 800–810 (2020).
Kim, J. S. et al. Characterization of degenerative human facet joints and facet joint capsular tissues. Osteoarthr. Cartil. 23 , 2242–2251 (2015).
Seidel, M. F., Netzer, C., Chobaz, V., Hugle, T. & Geurts, J. Localization of nerve growth factor expression to structurally damaged cartilaginous tissues in human lumbar facet joint osteoarthritis. Front. Immunol. 13 , 783076 (2022).
Dakin, P. et al. Efficacy and safety of fasinumab in patients with chronic low back pain: a phase II/III randomised clinical trial. Ann. Rheum. Dis. 80 , 509–517 (2020).
Andoh, T., Asakawa, Y. & Kuraishi, Y. Non-myelinated C-fibers, but not myelinated A-fibers, elongate into the epidermis in dry skin with itch. Neurosci. Lett. 672 , 84–89 (2018).
Eftekhari, S., Warfvinge, K., Blixt, F. W. & Edvinsson, L. Differentiation of nerve fibers storing CGRP and CGRP receptors in the peripheral trigeminovascular system. J. Pain 14 , 1289–1303 (2013).
Melo-Carrillo, A. et al. Fremanezumab-A humanized monoclonal anti-CGRP antibody-inhibits thinly myelinated (Adelta) but not unmyelinated (C) menin.geal nociceptors. J. Neurosci. 37 , 10587–10596 (2017).
Buma, P., Elmans, L., Van Den Berg, W. B. & Schrama, L. H. Neurovascular plasticity in the knee joint of an arthritic mouse model. Anat. Rec. 260 , 51–61 (2000).
Dong, T. et al. Calcitonin gene-related peptide can be selected as a predictive biomarker on progression and prognosis of knee osteoarthritis. Int. Orthop. 39 , 1237–1243 (2015).
Benschop, R. J. et al. Development of a novel antibody to calcitonin gene-related peptide for the treatment of osteoarthritis-related pain. Osteoarthr. Cartil. 22 , 578–585 (2014).
Jin, Y. et al. CGRP blockade by galcanezumab was not associated with reductions in signs and symptoms of knee osteoarthritis in a randomized clinical trial. Osteoarthr. Cartil. 26 , 1609–1618 (2018).
Mogil, J. S. Qualitative sex differences in pain processing: emerging evidence of a biased literature. Nat. Rev. Neurosci. 21 , 353–365 (2020).
Halievski, K., Ghazisaeidi, S. & Salter, M. W. Sex-dependent mechanisms of chronic pain: a focus on microglia and P2X4R. J. Pharmacol. Exp. Ther. 375 , 202–209 (2020).
Uchida, K. et al. Differential synovial CGRP/RAMP1 expression in men and women with knee osteoarthritis. Cureus. 13 , e15483 (2021).
Miller, R. J., Jung, H., Bhangoo, S. K. & White, F. A. Cytokine and chemokine regulation of sensory neuron function. Handb. Exp. Pharmacol. 194 , 417–449 (2009).
White, F. A., Feldman, P. & Miller, R. J. Chemokine signaling and the management of neuropathic pain. Mol. Interv. 9 , 188–195 (2009).
Decosterd, I. & Woolf, C. J. Spared nerve injury: an animal model of persistent peripheral neuropathic pain. Pain 87 , 149–158 (2000).
White, F. A. & Miller, R. J. Insights into the regulation of chemokine receptors by molecular signaling pathways: Functional roles in neuropathic pain. Brain Behav. Immun. 24 , 859–865 (2010).
Miller, R. E. et al. CCR2 chemokine receptor signaling mediates pain in experimental osteoarthritis. Proc. Natl. Acad. Sci. USA 109 , 20602–20607 (2012).
Griffith, J. W., Sokol, C. L. & Luster, A. D. Chemokines and chemokine receptors: positioning cells for host defense and immunity. Annu. Rev. Immunol. 32 , 659–702 (2014).
Raghu, H. et al. CCL2/CCR2, but not CCL5/CCR5, mediates monocyte recruitment, inflammation and cartilage destruction in osteoarthritis. Ann. Rheum. Dis. 76 , 914–922 (2017).
Ishihara, S. et al. The role of intra-articular neuronal CCR2 receptors in knee joint pain associated with experimental osteoarthritis in mice. Arthritis Res. Ther. 23 , 103 (2021).
Guan, Z. et al. Injured sensory neuron-derived CSF1 induces microglial proliferation and DAP12-dependent pain. Nat. Neurosci. 19 , 94–101 (2016).
Zhang, Z. J., Jiang, B. C. & Gao, Y. J. Chemokines in neuron-glial cell interaction and pathogenesis of neuropathic pain. Cell. Mol. Life Sci. 74 , 3275–3291 (2017).
Zhuang, Z. Y. et al. Role of the CX3CR1/p38 MAPK pathway in spinal microglia for the development of neuropathic pain following nerve injury-induced cleavage of fractalkine. Brain Behav. Immun. 21 , 642–651 (2007).
Grace, P. M., Hutchinson, M. R., Maier, S. F. & Watkins, L. R. Pathological pain and the neuroimmune interface. Nat. Rev. Immunol. 14 , 217–231 (2014).
Chen, G., Zhang, Y. Q., Qadri, Y. J., Serhan, C. N. & Ji, R. R. Microglia in pain: detrimental and protective roles in pathogenesis and resolution of pain. Neuron 100 , 1292–1311 (2018).
Watkins, L. R. et al. Glia as the “bad guys”: implications for improving clinical pain control and the clinical utility of opioids. Brain Behav. Immun. 21 , 131–146 (2007).
Chavan, S. S., Pavlov, V. A. & Tracey, K. J. Mechanisms and therapeutic relevance of neuro-immune communication. Immunity 46 , 927–942 (2017).
Scholz, J. & Woolf, C. J. The neuropathic pain triad: neurons, immune cells and glia. Nat. Neurosci. 10 , 1361–1368 (2007).
Ji, R. R., Chamessian, A. & Zhang, Y. Q. Pain regulation by non-neuronal cells and inflammation. Science 354 , 572–577 (2016).
Kawasaki, Y., Zhang, L., Cheng, J. K. & Ji, R. R. Cytokine mechanisms of central sensitization: distinct and overlapping role of interleukin-1beta, interleukin-6, and tumor necrosis factor-alpha in regulating synaptic and neuronal activity in the superficial spinal cord. J. Neurosci. 28 , 5189–5194 (2008).
Wojdasiewicz, P., Poniatowski, L. A. & Szukiewicz, D. The role of inflammatory and anti-inflammatory cytokines in the pathogenesis of osteoarthritis. Mediators Inflamm. 2014 , 561459 (2014).
Kloppenburg, M. et al. Etanercept in patients with inflammatory hand osteoarthritis (EHOA): a multicentre, randomised, double-blind, placebo-controlled trial. Ann. Rheum. Dis. 77 , 1757–1764 (2018).
Aitken, D. et al. A randomised double-blind placebo-controlled crossover trial of HUMira (adalimumab) for erosive hand OsteoaRthritis - the HUMOR trial. Osteoarthr. Cartil. 26 , 880–887 (2018).
Fu, W. et al. 14-3-3 epsilon is an intracellular component of TNFR2 receptor complex and its activation protects against osteoarthritis. Ann. Rheum. Dis. 80 , 1615–1627 (2021).
Pickar-Oliver, A. & Gersbach, C. A. The next generation of CRISPR-Cas technologies and applications. Nat. Rev. Mol. Cell Biol. 20 , 490–507 (2019).
Barrangou, R. et al. CRISPR provides acquired resistance against viruses in prokaryotes. Science 315 , 1709–1712 (2007).
Makarova, K. S. et al. An updated evolutionary classification of CRISPR–Cas systems. Nat. Rev. Microbiol 13 , 722–736 (2015).
Koonin, E. V., Makarova, K. S. & Zhang, F. Diversity, classification and evolution of CRISPR-Cas systems. Curr. Opin. Microbiol. 37 , 67–78 (2017).
Shmakov, S. et al. Diversity and evolution of class 2 CRISPR-Cas systems. Nat. Rev. Microbiol 15 , 169–182 (2017).
Hsu, P. D., Lander, E. S. & Zhang, F. Development and applications of CRISPR-Cas9 for genome engineering. Cell 157 , 1262–1278 (2014).
Jinek, M. et al. A programmable dual-RNA-guided DNA endonuclease in adaptive bacterial immunity. Science 337 , 816–821 (2012).
Wyman, C. & Kanaar, R. DNA double-strand break repair: all’s well that ends well. Annu. Rev. Genet. 40 , 363–383 (2006).
Bibikova, M., Golic, M., Golic, K. G. & Carroll, D. Targeted chromosomal cleavage and mutagenesis in Drosophila using zinc-finger nucleases. Genetics 161 , 1169–1175 (2002).
Cong, L. et al. Multiplex genome engineering using CRISPR/Cas systems. Science 339 , 819–823 (2013).
Gutschner, T., Haemmerle, M., Genovese, G., Draetta, G. F. & Chin, L. Post-translational regulation of Cas9 during G1 enhances homology-directed repair. Cell Rep. 14 , 1555–1566 (2016).
Pinder, J., Salsman, J. & Dellaire, G. Nuclear domain ‘knock-in’ screen for the evaluation and identification of small molecule enhancers of CRISPR-based genome editing. Nucleic Acids Res. 43 , 9379–9392 (2015).
Niu, Y. et al. Generation of gene-modified cynomolgus monkey via Cas9/RNA-mediated gene targeting in one-cell embryos. Cell 156 , 836–843 (2014).
Steven, L., Staahl, B. T., Alla, R. K. & Doudna, J. A. Enhanced homology-directed human genome engineering by controlled timing of CRISPR/Cas9 delivery. eLife 3 , e04766 (2014).
Voytas, D. F. & Gao, C. Precision genome engineering and agriculture: opportunities and regulatory challenges. PLoS Biol. 12 , e1001877 (2014).
Lambert, L. J. et al. Increased trabecular bone and improved biomechanics in an osteocalcin-null rat model created by CRISPR/Cas9 technology. Dis. Model Mech. 9 , 1169–1179 (2016).
Matsukawa, A. & Yoshinaga, M. Sequential generation of cytokines during the initiative phase of inflammation, with reference to neutrophils. Inflamm. Res. 47 , S137–S144 (1998).
Cigan, A. D. et al. High seeding density of human chondrocytes in agarose produces tissue-engineered cartilage approaching native mechanical and biochemical properties. J. Biomech. 49 , 1909–1917 (2016).
Karlsen, T. A., Pernas, P. F., Staerk, J., Caglayan, S. & Brinchmann, J. E. Generation of IL1β-resistant chondrocytes using CRISPR-CAS genome editing. Osteoarthr. Cartil . 24 , S325(2016).
Eyquem, J. et al. Targeting a CAR to the TRAC locus with CRISPR/Cas9 enhances tumour rejection. Nature 543 , 113–117 (2017).
Ran, F. A. et al. In vivo genome editing using Staphylococcus aureus Cas9. Nature 520 , 186–191 (2015).
Ho, B. X., Loh, S. J. H., Chan, W. K. & Soh, B. S. In vivo genome editing as a therapeutic approach. Int. J. Mol. Sci. 19 , 3721 (2018).
Chevalier, X., Eymard, F. & Richette, P. Biologic agents in osteoarthritis: hopes and disappointments. Nat. Rev. Rheumatol. 9 , 400–410 (2013).
Hellio Le Graverand-Gastineau, M. P. OA clinical trials: current targets and trials for OA. Choosing molecular targets: what have we learned and where we are headed? Osteoarthr. Cartil. 17 , 1393–1401 (2009).
Seidel, M. F., Wise, B. L. & Lane, N. E. Nerve growth factor: an update on the science and therapy. Osteoarthr. Cartil. 21 , 1223–1228 (2013).
Zhao, L. et al. Exploration of CRISPR/Cas9-based gene editing as therapy for osteoarthritis. Ann. Rheum. Dis. 78 , 676–682 (2019).
Enache, O. M. et al. Cas9 activates the p53 pathway and selects for p53-inactivating mutations. Nat. Genet. 52 , 662–668 (2020).
Kosicki, M., Tomberg, K. & Bradley, A. Repair of double-strand breaks induced by CRISPR-Cas9 leads to large deletions and complex rearrangements. Nat. Biotechnol. 36 , 765–771 (2018).
Xu, S. et al. CAS9 is a genome mutator by directly disrupting DNA-PK dependent DNA repair pathway. Protein Cell 11 , 352–365 (2020).
Abudayyeh, O. O. et al. RNA targeting with CRISPR-Cas13. Nature 550 , 280–284 (2017).
Xu, C. et al. Programmable RNA editing with compact CRISPR-Cas13 systems from uncultivated microbes. Nat. Methods 18 , 499–506 (2021).
Zeballos, C. M. & Gaj, T. Next-Generation CRISPR Technologies and Their Applications in Gene and Cell Therapy. Trends Biotechnol. 39 , 692–705 (2021).
He, B. et al. Modulation of metabolic functions through Cas13d-mediated gene knockdown in liver. Protein Cell 11 , 518–524 (2020).
Zhou, C. et al. CasRx-mediated RNA targeting prevents choroidal neovascularization in a mouse model of age-related macular degeneration. Natl. Sci. Rev. 7 , 835–837 (2020).
Lin, A. C. et al. Modulating hedgehog signaling can attenuate the severity of osteoarthritis. Nat. Med. 15 , 1421–1425 (2009).
Razzaque, M. S., Soegiarto, D. W., Chang, D., Long, F. & Lanske, B. Conditional deletion of Indian hedgehog from collagen type 2alpha1-expressing cells results in abnormal endochondral bone formation. J. Pathol. 207 , 453–461 (2005).
Wu, Z., Shou, L., Wang, J. & Xu, X. Identification of the key gene and pathways associated with osteoarthritis via single-cell RNA sequencing on synovial fibroblasts. Medicine 99 , e21707 (2020).
Ji, Q. et al. Single-cell RNA-seq analysis reveals the progression of human osteoarthritis. Ann. Rheum. Dis. 78 , 100–110 (2019).
Li, C. et al. Single cell sequencing revealed the underlying pathogenesis of the development of osteoarthritis. Gene 757 , 144939 (2020).
Maes, C. et al. Osteoblast precursors, but not mature osteoblasts, move into developing and fractured bones along with invading blood vessels. Dev. Cell 19 , 329–344 (2010).
Mizoguchi, T. et al. Osterix marks distinct waves of primitive and definitive stromal progenitors during bone marrow development. Dev. Cell 29 , 340–349 (2014).
Mizuhashi, K. et al. Resting zone of the growth plate houses a unique class of skeletal stem cells. Nature 563 , 254–258 (2018).
Ono, N. et al. Vasculature-associated cells expressing nestin in developing bones encompass early cells in the osteoblast and endothelial lineage. Dev. Cell 29 , 330–339 (2014).
Wang, T. et al. Specific deletion of beta-Catenin in Col2-expressing cells leads to defects in epiphyseal bone. Int. J. Biol. Sci. 13 , 1540–1546 (2017).
Kuang, D. Y. et al. Complete chloroplast genome sequence of Magnolia kwangsiensis (Magnoliaceae): implication for DNA barcoding and population genetics. Genome 54 , 663–673 (2011).
Hsu, C. M., de Palmas, S., Kuo, C. Y., Denis, V. & Chen, C. A. Identification of scleractinian coral recruits using fluorescent censusing and DNA barcoding techniques. PLoS One 9 , e107366 (2014).
McKenna, A. et al. Whole-organism lineage tracing by combinatorial and cumulative genome editing. Science 353 , aaf7907 (2016).
McKenna, A. & Gagnon, J. A. Recording development with single cell dynamic lineage tracing. Development 146 (2019).
Wagner, D. E. & Klein, A. M. Lineage tracing meets single-cell omics: opportunities and challenges. Nat. Rev. Genet. 21 , 410–427 (2020).
Kebschull, J. M. & Zador, A. M. Cellular barcoding: lineage tracing, screening and beyond. Nat. Methods 15 , 871–879 (2018).
Galluzzi, L. et al. Molecular mechanisms of cell death: recommendations of the Nomenclature Committee on Cell Death 2018. Cell Death Differ. 25 , 486–541 (2018).
Ameisen, J. C. On the origin, evolution, and nature of programmed cell death: a timeline of four billion years. Cell Death Differ. 9 , 367–393 (2002).
Elmore, S. Apoptosis: a review of programmed cell death. Toxicol. Pathol. 35 , 495–516 (2007).
Fuchs, Y. & Steller, H. Programmed cell death in animal development and disease. Cell 147 , 742–758 (2011).
Ouyang, L. et al. Programmed cell death pathways in cancer: a review of apoptosis, autophagy and programmed necrosis. Cell Prolif. 45 , 487–498 (2012).
Bedoui, S., Herold, M. J. & Strasser, A. Emerging connectivity of programmed cell death pathways and its physiological implications. Nat. Rev. Mol. Cell Biol. 21 , 678–695 (2020).
D’Souza, C. A. & Heitman, J. Dismantling the Cryptococcus coat. Trends Microbiol 9 , 112–113 (2001).
Broz, P. & Dixit, V. M. Inflammasomes: mechanism of assembly, regulation and signalling. Nat. Rev. Immunol. 16 , 407–420 (2016).
He, W. T. et al. Gasdermin D is an executor of pyroptosis and required for interleukin-1beta secretion. Cell Res. 25 , 1285–1298 (2015).
de Gassart, A. & Martinon, F. Pyroptosis: caspase-11 unlocks the gates of death. Immunity 43 , 835–837 (2015).
Kovacs, S. B. & Miao, E. A. Gasdermins: effectors of pyroptosis. Trends Cell Biol. 27 , 673–684 (2017).
Liu, X. et al. Inflammasome-activated gasdermin D causes pyroptosis by forming membrane pores. Nature 535 , 153–158 (2016).
Wu, X., Ren, G., Zhou, R., Ge, J. & Chen, F. H. The role of Ca(2+) in acid-sensing ion channel 1a-mediated chondrocyte pyroptosis in rat adjuvant arthritis. Lab. Investig. 99 , 499–513 (2019).
Zhang, Y., Lin, Z., Chen, D. & He, Y. CY-09 attenuates the progression of osteoarthritis via inhibiting NLRP3 inflammasome-mediated pyroptosis. Biochem. Biophys. Res. Commun. 553 , 119–125 (2021).
Hu, J. et al. Loganin ameliorates cartilage degeneration and osteoarthritis development in an osteoarthritis mouse model through inhibition of NF-kappaB activity and pyroptosis in chondrocytes. J. Ethnopharmacol. 247 , 112261 (2020).
Zhang, L. et al. Increased HIF-1alpha in knee osteoarthritis aggravate synovial fibrosis via fibroblast-like synoviocyte pyroptosis. Oxid. Med. Cell Longev. 2019 , 6326517 (2019).
Xie, Y. et al. Ferroptosis: process and function. Cell Death Differ. 23 , 369–379 (2016).
Li, J. et al. Ferroptosis: past, present and future. Cell Death Dis. 11 , 88 (2020).
Dixon, S. J. et al. Ferroptosis: an iron-dependent form of nonapoptotic cell death. Cell 149 , 1060–1072 (2012).
Imai, H., Matsuoka, M., Kumagai, T., Sakamoto, T. & Koumura, T. Lipid peroxidation-dependent cell death regulated by GPx4 and ferroptosis. Curr. Top. Microbiol. Immunol. 403 , 143–170 (2017).
Yang, W. S. & Stockwell, B. R. Ferroptosis: death by lipid peroxidation. Trends Cell Biol. 26 , 165–176 (2016).
Jiang, X., Stockwell, B. R. & Conrad, M. Ferroptosis: mechanisms, biology and role in disease. Nat. Rev. Mol. Cell Biol. 22 , 266–282 (2021).
Stockwell, B. R., Jiang, X. & Gu, W. Emerging mechanisms and disease relevance of ferroptosis. Trends Cell Biol. 30 , 478–490 (2020).
Mobasheri, A. et al. The role of metabolism in the pathogenesis of osteoarthritis. Nat. Rev. Rheumatol. 13 , 302–311 (2017).
Robinson, W. H. et al. Low-grade inflammation as a key mediator of the pathogenesis of osteoarthritis. Nat. Rev. Rheumatol. 12 , 580–592 (2016).
Yao, X. et al. Chondrocyte ferroptosis contribute to the progression of osteoarthritis. J. Orthop. Translat. 27 , 33–43 (2021).
Wang, S. et al. Mechanical overloading induces GPX4-regulated chondrocyte ferroptosis in osteoarthritis via Piezo1 channel facilitated calcium influx. J. Adv. Res. (2022).
Wu, J. et al. Intercellular interaction dictates cancer cell ferroptosis via NF2-YAP signalling. Nature 572 , 402–406 (2019).
Yang, W. H. et al. The Hippo pathway effector TAZ regulates ferroptosis in renal cell carcinoma. Cell Rep. 28 , 2501–2508 e2504 (2019).
Yang, W. H. et al. The Hippo pathway effector YAP promotes ferroptosis via the E3 ligase SKP2. Mol. Cancer Res. 19 , 1005–1014 (2021).
Lee, H. et al. Energy-stress-mediated AMPK activation inhibits ferroptosis. Nat. Cell Biol. 22 , 225–234 (2020).
Li, C. et al. LKB1-AMPK axis negatively regulates ferroptosis by inhibiting fatty acid synthesis. Signal Transduct. Target Ther. 5 , 187 (2020).
Codogno, P., Mehrpour, M. & Proikas-Cezanne, T. Canonical and non-canonical autophagy: variations on a common theme of self-eating? Nat. Rev. Mol. Cell Biol. 13 , 7–12 (2011).
Nakamura, S. & Yoshimori, T. New insights into autophagosome-lysosome fusion. J. Cell Sci. 130 , 1209–1216 (2017).
Alers, S., Loffler, A. S., Wesselborg, S. & Stork, B. Role of AMPK-mTOR-Ulk1/2 in the regulation of autophagy: cross talk, shortcuts, and feedbacks. Mol. Cell. Biol. 32 , 2–11 (2012).
Moruno-Manchon, J. F., Perez-Jimenez, E. & Knecht, E. Glucose induces autophagy under starvation conditions by a p38 MAPK-dependent pathway. Biochem. J. 449 , 497–506 (2013).
Qin, L., Wang, Z., Tao, L. & Wang, Y. ER stress negatively regulates AKT/TSC/mTOR pathway to enhance autophagy. Autophagy 6 , 239–247 (2010).
Carames, B., Taniguchi, N., Otsuki, S., Blanco, F. J. & Lotz, M. Autophagy is a protective mechanism in normal cartilage, and its aging-related loss is linked with cell death and osteoarthritis. Arthritis Rheumatol. 62 , 791–801 (2010).
López de Figueroa, P., Lotz, M. K., Blanco, F. J. & Caramés, B. Autophagy activation and protection from mitochondrial dysfunction in human chondrocytes. Arthritis Rheumatol. 67 , 966–976 (2015).
Zhang, Y. et al. Cartilage-specific deletion of mTOR upregulates autophagy and protects mice from osteoarthritis. Ann. Rheum. Dis. 74 , 1432–1440 (2015).
Takayama, K. et al. Local intra-articular injection of rapamycin delays articular cartilage degeneration in a murine model of osteoarthritis. Arthritis Res. Ther. 16 , 482 (2014).
Zawieja, D. Lymphatic biology and the microcirculation: past, present and future. Microcirculation 12 , 141–150 (2015).
Wilting, J., Becker, J., Buttler, K. & Weich, H. A. Lymphatics and inflammation. Curr. Med. Chem. 16 , 4581–4592 (2009).
Fei, Z. et al. Akt/Protein kinase B is required for lymphatic network formation, remodeling, and valve development. Am. J. Pathol. 177 , 2124–2133 (2010).
van der Flier, A. et al. Endothelial alpha5 and alphav integrins cooperate in remodeling of the vasculature during development. Development 137 , 2439–2449 (2010).
Kenney, H. M. et al. Lineage tracing reveals evidence of a popliteal lymphatic muscle progenitor cell that is distinct from skeletal and vascular muscle progenitors. Sci. Rep. 10 , 18088 (2020).
Van Helden, D. F. Pacemaker potentials in lymphatic smooth muscle of the guinea-pig mesentery. J. Physiol. 471 , 465–479 (1993).
von der Weid, P. Y. & Zawieja, D. C. Lymphatic smooth muscle: the motor unit of lymph drainage. Int. J. Biochem. Cell Biol. 36 , 1147–1153 (2004).
Guo, R. et al. Inhibition of lymphangiogenesis and lymphatic drainage via vascular endothelial growth factor receptor 3 blockade increases the severity of inflammation in a mouse model of chronic inflammatory arthritis. Arthritis Rheumatol . 60 , 2666–2676 (2010).
Li, J. et al. Efficacy of B cell depletion therapy for murine joint arthritis flare is associated with increased lymphatic flow. Arthritis Rheumatol. 65 , 130–138 (2013).
Li, J., Quan, Z., Wood, R. W., Kuzin, I. & Schwarz, E. M. CD23/CD21B-cell translocation and ipsilateral lymph node collapse is associated with asymmetric arthritic flare in TNF-Tg mice. Arthritis Res. Ther. 13 , R138 (2011).
Liang, Q. et al. Lymphatic endothelial cells efferent to inflamed joints produce iNOS and inhibit lymphatic vessel contraction and drainage in TNF-induced arthritis in mice. Arthritis Res. Ther. 18 , 62 (2016).
Zhou, Q. et al. Vascular endothelial growth factor C attenuates joint damage in chronic inflammatory arthritis by accelerating local lymphatic drainage in mice. Arthritis Rheumatol. 63 , 2318–2328 (2011).
Zhou, Q., Wood, R., Schwarz, E. M., Wang, Y. J. & Xing, L. Near-infrared lymphatic imaging demonstrates the dynamics of lymph flow and lymphangiogenesis during the acute versus chronic phases of arthritis in mice. Arthritis Rheumatol. 62 , 1881–1889 (2014).
Shi, J. et al. Distribution and alteration of lymphatic vessels in knee joints of normal and osteoarthritic mice. Arthritis Rheumatol. 66 , 657–666 (2014).
Shi, J. X. et al. Use of a whole-slide imaging system to assess the presence and alteration of lymphatic vessels in joint sections of arthritic mice. Biotech. Histochem. 88 , 428–439 (2013).
Li, J. et al. Expanded CD23(+)/CD21(hi) B cells in inflamed lymph nodes are associated with the onset of inflammatory-erosive arthritis in TNF-transgenic mice and are targets of anti-CD20 therapy. J. Immunol. 184 , 6142–6150 (2010).
Bouta, E. M. et al. Targeting lymphatic function as a novel therapeutic intervention for rheumatoid arthritis. Nat. Rev. Rheumatol. 14 , 94–106 (2018).
Bell, R. D. et al. Altered lymphatic vessel anatomy and markedly diminished lymph clearance in affected hands of patients with active rheumatoid arthritis. Arthritis Rheumatol. 72 , 1447–1455 (2020).
Albuquerque, M. & de Lima, J. P. Articular lymphoscintigraphy in human knees using radiolabeled dextran. Lymphology 23 , 215–218 (1990).
Walsh, D. A. et al. Lymphatic vessels in osteoarthritic human knees. Osteoarthr. Cartil. 20 , 405–412 (2012).
Mesquita, S. D. et al. Functional aspects of meningeal lymphatics in ageing and Alzheimer’s disease. Nature 82 , 185–191 (2018).
Shaw, T., Nixon, J. S. & Bottomley, K. M. Metalloproteinase inhibitors: new opportunities for the treatment of rheumatoid arthritis and osteoarthritis. Expert Opin. Investig. Drugs 9 , 1469–1478 (2000).
Krzeski, P. et al. Development of musculoskeletal toxicity without clear benefit after administration of PG-116800, a matrix metalloproteinase inhibitor, to patients with knee osteoarthritis: a randomized, 12-month, double-blind, placebo-controlled study. Arthritis Res. Ther. 9 , 1–11 (2007).
Zhang, Y. et al. Activation of vascular endothelial growth factor receptor-3 in macrophages restrains TLR4-NF-κB signaling and protects against endotoxin shock. Immunity 40 , 501–514 (2014).
Shen, J. et al. Deletion of the type II TGF-β receptor gene in articular chondrocytes leads to progressive OA-like phenotype in mice. Arthritis Rheum. 65 , 3107–3119 (2013).
Xia, C. et al. Activation of β-catenin in Col2-expressing chondrocytes leads to osteoarthritis-like defects in hip joint. J. Cell Physiol 234 , 18535–18543 (2019).
Lin, Xi. Impact of macrophages on the synovial lymphatic system in mice with posttraumatic osteoarthritis. PhD dissertation, University of Rochester (2020).
Download references
Acknowledgements
This work has been supported by the National Natural Science Foundation of China (NSFC) grants (82030067, 82161160342, and 82172397) to D.C. and L.T. and a grant from the Youth Innovation Promotion Association of the Chinese Academy of Sciences (2020353) to L.T. This work was also supported by the National Key Research and Development Program of China (2021YFB3800800 to L.T. and D.C). This work was also supported by the research grant NIH AG0599775. The data in Fig. 6 were generated by Dr. Xi Lin, a postdoctoral fellow at the University of Rochester Medical Center.
Author information
Authors and affiliations.
Research Center for Computer-aided Drug Discovery, Shenzhen Institute of Advanced Technology, Chinese Academy of Sciences, Shenzhen, 518005, China
Liping Tong, Huan Yu, Xingyun Huang & Di Chen
Faculty of Pharmaceutical Sciences, Shenzhen Institute of Advanced Technology, Chinese Academy of Sciences, Shenzhen, 518055, China
Huan Yu, Xingyun Huang & Di Chen
Department of Orthopedic Surgery, School of Medicine, Washington University in St. Louis, St. Louis, MO, 63110, USA
School of Medicine, Southern University of Science and Technology, Shenzhen, 518055, China
Guozhi Xiao
Department of Wound Repair and Rehabilitation, State Key Laboratory of Trauma, Burns and Combined Injury, Daping Hospital, Army Medical University, Chongqing, 400042, China
Research Center for Human Tissues and Organs Degeneration, Shenzhen Institute of Advanced Technology, Chinese Academy of Sciences, Shenzhen, 518055, China
Huaiyu Wang
Department of Pathology and Laboratory of Medicine, Center for Musculoskeletal Research, University of Rochester Medical Center, Rochester, NY, 14642, USA
Lianping Xing
You can also search for this author in PubMed Google Scholar
Contributions
Conceptualization, preparation and revision of the manuscript, D.C.; methodology, L.T., H.Y., L.C., and G.X.; preparing the manuscript, L.T., H.Y., X.H., J.S., L.X. and H.W. All authors have read and agreed to the published version of the manuscript.
Corresponding author
Correspondence to Di Chen .
Ethics declarations
Competing interests.
The authors declare no competing interests.
Rights and permissions
Open Access This article is licensed under a Creative Commons Attribution 4.0 International License, which permits use, sharing, adaptation, distribution and reproduction in any medium or format, as long as you give appropriate credit to the original author(s) and the source, provide a link to the Creative Commons license, and indicate if changes were made. The images or other third party material in this article are included in the article’s Creative Commons license, unless indicated otherwise in a credit line to the material. If material is not included in the article’s Creative Commons license and your intended use is not permitted by statutory regulation or exceeds the permitted use, you will need to obtain permission directly from the copyright holder. To view a copy of this license, visit http://creativecommons.org/licenses/by/4.0/ .
Reprints and permissions
About this article
Cite this article.
Tong, L., Yu, H., Huang, X. et al. Current understanding of osteoarthritis pathogenesis and relevant new approaches. Bone Res 10 , 60 (2022). https://doi.org/10.1038/s41413-022-00226-9
Download citation
Received : 04 April 2022
Revised : 27 May 2022
Accepted : 19 June 2022
Published : 20 September 2022
DOI : https://doi.org/10.1038/s41413-022-00226-9
Share this article
Anyone you share the following link with will be able to read this content:
Sorry, a shareable link is not currently available for this article.
Provided by the Springer Nature SharedIt content-sharing initiative
This article is cited by
A dna tetrahedron-based ferroptosis-suppressing nanoparticle: superior delivery of curcumin and alleviation of diabetic osteoporosis.
- Zhengwen Cai
- Yunfeng Lin
Bone Research (2024)
Phototherapy techniques for the management of musculoskeletal disorders: strategies and recent advances
- Zhenhe Zhang
Biomaterials Research (2023)
Expression pattern analysis of m6A regulators reveals IGF2BP3 as a key modulator in osteoarthritis synovial macrophages
- Hongbo Zhang
- Daozhang Cai
Journal of Translational Medicine (2023)
Resatorvid alleviates experimental inflammatory TMJOA by restraining chondrocyte pyroptosis and synovial inflammation
Arthritis Research & Therapy (2023)
Risk of metabolic abnormalities in osteoarthritis: a new perspective to understand its pathological mechanisms
- Guizheng Wei
Bone Research (2023)
Quick links
- Explore articles by subject
- Guide to authors
- Editorial policies

- Reference Manager
- Simple TEXT file
People also looked at
Review article, exercise for osteoarthritis: a literature review of pathology and mechanism.
- 1 College of Kinesiology, Shenyang Sport University, Shenyang, China
- 2 Department of Sport Rehabilitation, Shanghai University of Sport, Shanghai, China
- 3 Department of Rehabilitation Medicine, Shanghai Shangti Orthopedic Hospital, Shanghai, China
Osteoarthritis (OA) has a very high incidence worldwide and has become a very common joint disease in the elderly. Currently, the treatment methods for OA include surgery, drug therapy, and exercise therapy. In recent years, the treatment of certain diseases by exercise has received increasing research and attention. Proper exercise can improve the physiological function of various organs of the body. At present, the treatment of OA is usually symptomatic. Limited methods are available for the treatment of OA according to its pathogenesis, and effective intervention has not been developed to slow down the progress of OA from the molecular level. Only by clarifying the mechanism of exercise treatment of OA and the influence of different exercise intensities on OA patients can we choose the appropriate exercise prescription to prevent and treat OA. This review mainly expounds the mechanism that exercise alleviates the pathological changes of OA by affecting the degradation of the ECM, apoptosis, inflammatory response, autophagy, and changes of ncRNA, and summarizes the effects of different exercise types on OA patients. Finally, it is found that different exercise types, exercise intensity, exercise time and exercise frequency have different effects on OA patients. At the same time, suitable exercise prescriptions are recommended for OA patients.
Introduction
Osteoarthritis (OA) has a very high incidence worldwide, and it is strongly associated with age ( van Saase et al., 1989 ). Most of the patients with OA are aged over 60. With the aging of the population, the disease has become a common joint disease today. Heredity, hormones, diet, obesity, smoking, and drinking can lead to OA ( Felson et al., 1997 ). Its early symptoms mainly include pain and joint stiffness, and later secondary changes such as muscle atrophy and joint contracture occur ( Bennell et al., 2012 ). Therefore, we advocate early intervention for patients with OA to prevent further deterioration of the disease. However, the disease treatment still encounters many shortcomings, and further research and exploration are needed. Currently, the treatment methods for OA include surgery, drug therapy, and exercise therapy ( Spahn et al., 2013 ). In recent years, the treatment of certain diseases by exercise has received increasing research and attention. Exercise is an economical and effective treatment ( Ettinger et al., 1997 ). Proper exercise can improve the physiological function of various organs of the body and improve the overall morphology of the body ( Benedetti et al., 2018 ). In addition, exercise can also relieve pain ( Belavy et al., 2021 ; Zheng et al., 2021 ; Peng et al., 2022 ; Wu et al., 2022 ). For OA patients, ladder treatment is generally adopted, starting with basic treatment. If it is ineffective, drug therapy or surgery can be used, and exercise therapy is one of the basic treatment methods. The effects of different exercise types on OA have been widely studied.
At present, the treatment of OA is usually symptomatic. Limited methods are available for the treatment of OA according to its pathogenesis. Exercise can alleviate OA at a molecular level. Only by clarifying the mechanism of exercise treatment of OA and the influence of different exercise intensities on normal joints and OA patients can we choose the appropriate exercise prescription to prevent and treat OA. This review mainly expounds the mechanism that exercise alleviates the pathological changes of OA by affecting the degradation of the ECM, apoptosis, inflammatory response, autophagy, and changes of ncRNA, and summarizes the effects of different exercise types on OA patients. Finally, it is found that different exercise types, exercise intensity, exercise time and exercise frequency have different effects on OA patients. At the same time, suitable exercise prescriptions are recommended for OA patients.
Pathological Change Mechanism of Osteoarthritis
For OA patients, the integrity of the entire joint tissue is damaged, including articular cartilage, subchondral bone, and synovial membrane ( Loeser et al., 2012 ). Articular cartilage is mainly composed of the extracellular matrix (ECM), consisting of water, collagen, proteoglycans, mucopolysaccharides, type II collagen, and chondrocytes ( Luo et al., 2017 ). With the presence of prolonged, excessive mechanical stimulation of the body, articular cartilage will suffer. In the early stage of articular cartilage injury, the concentration of growth factors in the ECM increases as chondrocytes gather in the damaged area, resulting in transient cell proliferation and ECM synthesis ( Suri and Walsh, 2012 ). As the damage worsens, the blood supply to the articular cartilage worsens to the point that the cartilage does not have adequate access to nutrients. This condition results in cartilage cell apoptosis, ECM synthesis, and the disappearance of the articular cartilage degeneration ( Eyre, 2004 ). The two bones constantly rub, resulting in joint pain, swelling, and function limitation. Its continuous development causes OA ( Rim et al., 2020 ). Subchondral bone includes subchondral cancellous bone and cortical plates. X-ray and Magnetic Resonance Imaging (MRI) diagnosis can reveal the abnormal remodeling of subchondral bone and a series of changes in bonemorphology, such as bone spurs, osteophytes, and wear in OA patients ( Braun and Gold, 2012 ). This sequence of changes may be caused by an imbalance in the production and destruction of osteoblasts and osteoclasts ( Burr and Gallant, 2012 ). This morphological change of subchondral bone may also cause damage to the articular cartilage overlying it. Patients with OA have an exceptionally high probability of suffering from synovitis, and this condition is related to the pain and function of the knee joint during OA development ( Sowers et al., 2011 ). The synovial membrane is the connective tissue membrane covered on the inner surface of the joint capsule, which can produce synovial fluid to reduce joint friction and the loss of cartilage and ensure the metabolism and nutrition supply of joint cartilage ( Pap et al., 2020 ). Synovial cells mainly include synovial macrophages, fibroblast-like synovial cells, and mesenchymal stem cells. The macrophages can engulf the damaged tissue. When synovitis occurs in the body, the macrophages decompose to produce inflammatory factors, and the generation and degradation of cartilage matrix are in dynamic balance under normal conditions. Inflammatory mediators can destroy this balance, decompose chondrocytes, and aggravate synovitis ( Da et al., 2007 ). Fibroblast-like synovial cells can produce hyaluronic acid, which is an essential component of joint synovial fluid. During OA progression, the content of hyaluronic acid is remarkably reduced, resulting in the reduction of joint function and damage to the integrity of the joint surface ( Scanzello and Goldring, 2012 ).
Above all, articular cartilage, synovium, and subchondral bone abnormalities are the main pathological changes of OA ( Valdes and Spector, 2010 ). Considering that articular cartilage receives nutrients through synovial fluid, articular cartilage in patients with synovitis may undergo pathological changes. Moreover, the increase of macrophages in patients with synovitis may cause subchondral bones in OA to form osteophytes. Ayral et al. (2005) evaluated the correlation between synovitis and the severity of cartilage structure damage in OA patients through a one-year multicenter longitudinal study involving 422 patients. After arthroscopic and pain scoring, they found that compared with patients with normal synovial membrane, patients with synovitis had more severe articular cartilage lesions. The degree of deterioration after 1 year was statistically different. Yusup et al. (2015) studied 40 patients with knee OA and scored the structures such as joint cartilage, subchondral bone, and synovial membrane by using 3.0-T MRI and found that synovitis mainly occurred in the early and late stage of OA patients. The destruction of joint cartilage and a series of changes in subchondral bone could induce synovitis, and a strong correlation was observed among the three parameters. Some studies ( Norrdin et al., 1998 ) use horse as a model to study the pathological changes of OA, in which the morphological changes of subchondral bone occur before articular cartilage injury. In other studies ( Brandt et al., 1991 ), dog is used as the model to investigate the pathological changes of OA, in which the morphological changes of early subchondral bone coincided with an articular cartilage injury. Generally, the pathological mechanisms of articular cartilage, synovium, and subchondral bone in OA mainly include the degradation of ECM, cell proliferation, cell apoptosis, inflammatory reaction, changes of non-coding RNA, and methylation ( Mobasheri et al., 2017 ) ( Figure 1 ).
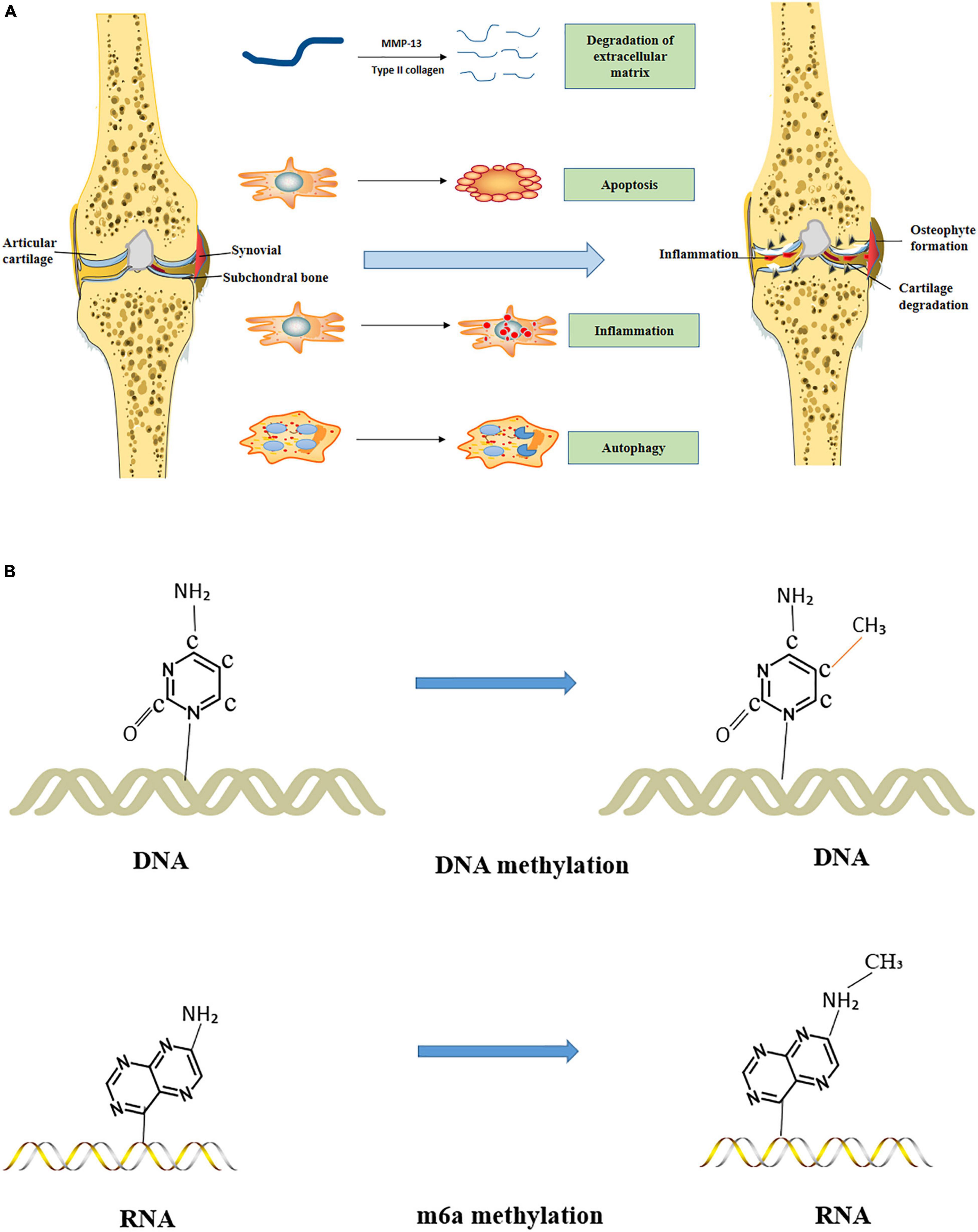
Figure 1. Pathogenesis of Osteoarthritis (OA). (A) The degradation of ECM, apoptosis, inflammatory response, and autophagy mechanisms in OA. (B) Methylation in OA.
Degradation of Extracellular Matrix
The ECM is a complex network of large molecules such as polysaccharides and proteins around cells. This structure and special cells make up the cartilage ( Theocharis et al., 2019 ). Many diseases are associated with changes in the composition and properties of ECM, such as OA. In healthy articular cartilage, the synthesis and metabolism of ECM should always maintain a dynamic balance to maintain homeostasis. The secretion and operation of some components of ECM are completed by articular cartilage ( Rahmati et al., 2017 ). When the activities of synthesis and catabolism of articular cartilage are imbalanced, the details and homeostasis of ECM are also affected ( Guo et al., 2002 ). Considering that the degradation of ECM leads to the loss of cartilage tissue, ECM also plays a role in maintaining the function of chondrocytes. The continuous degradation of ECM will induce OA.
Based on the summarizing of articles related to the pathogenesis of OA and the function of chondrocytes, Goldring and Goldring (2007) found that the degradation of ECM and decomposition of collagen could lead to the gradual loss of the shape and position of articular cartilage to induce OA. Setayeshmehr et al. (2019) summarized the functions and effects of various ECM scaffolds through summarizing and analyzing the related articles on hybrid and composite scaffolds derived from chondrocyte ECM. They concluded that the degradation of chondrocyte ECM could lead to cartilage degeneration. Malemud (2017) analyzed the role of matrix metalloproteinases in OA. They found that in the ECM of the cartilage, some essential proteins would degrade. Matrix metalloproteinases can promote or inhibit the degradation of these proteins, change the biomechanical properties of tissues, and destroy articular cartilage. In addition, based on the analysis of the action mechanism of ECM and its degrading enzymes in OA, the ECM of cartilage in OA patients can release certain fibronectin, thereby inducing the expression of related proteases and degrading the components of the ECM ( Pérez-García et al., 2019 ).
Kapoor et al. (2011) and Theocharis et al. (2019) also found that the tissue protein would be hydrolyzed gradually during the development of OA. Then, some catabolic mediators will be produced, which will induce the expression of some related cytokines and proteases, and finally lead to ECM degradation. This situation will lead to prolonged OA. Based on the analysis of the changes of some macromolecules in the ECM of early OA patients, the content of proteins related to ECM was changed, such as collagen and cartilage protein. Hence, the degradation of ECM and even the deterioration of OA disease occurred ( Lorenzo et al., 2004 ).
Apoptosis is an active gene-determined process that automatically ends life, and it is often called programmed cell death, a basic biological phenomenon of cells ( Musumeci et al., 2011 ). Apoptosis involves endogenous and exogenous pathways, which play an essential role in maintaining the function of various tissues in the body ( Elmore, 2007 ). A correlation has been observed between the degree of cartilage injury and apoptosis, which is a vital mechanism of cartilage injury ( Hwang and Kim, 2015 ). Moreover, the apoptosis of OA chondrocytes is related to cartilage degradation ( Kim et al., 2003 ). When OA occurs, it will produce matrix-degrading enzymes, leading to the degradation of the ECM and the destruction of cell homeostasis. Cell stress induces an oxidation reaction, leading to the apoptosis of chondrocytes and regulating the pathological changes of cartilage tissues ( Bauer et al., 2006 ).
Histologically, Musumeci et al. (2011) studied the articular cartilage of normal individuals and OA patients by staining apoptotic cells. They found that the articular cartilage of OA patients changed in structure. The pro-apoptotic receptor on the cell surface could induce apoptosis through an exogenous pathway in the damaged articular cartilage. Aigner et al. (2004) summarized the incidence, induction mechanism, and morphology of apoptosis in OA patients and found that apoptosis occurred in patients with OA, but the incidence was not high. The body induced apoptosis through specific proteoglycans, signaling molecules, and other pathways to regulate the pathological changes of articular cartilage and accelerate or delay the progression of OA. Based on the synthesis of related articles on OA and apoptosis, some articles indicate that apoptosis induces OA, while other articles indicate that OA induces apoptosis. These two views are disputed, and more reports believe that these conditions affect and promote each other and have a correlation ( Zamli and Sharif, 2011 ). Chondrocytes in the knee joints of patients with OA and the apoptosis of chondrocytes and its relationship with cartilage degradation have been studied. Notably, the findings indicate that first, in some populations, the morphology and function of cartilage are normal ( Hashimoto et al., 1998 ). Still, many apoptotic cells appear, indicating that apoptosis may lead to degenerative changes in articular cartilage, thus inducing OA. Furthermore, the number of apoptotic articular cartilage cells in the OA population increased, and the number of apoptotic cells was related to cartilage degradation. Finally, when all subjects, including the average population and OA patients, were analyzed, a correlation was found between age and apoptosis of chondrocytes. Similarly, rabbit chondrocytes have been studied and compared with mature rabbits. Results show that the cell density of each layer of articular cartilage in rabbits decreased, and the expression level of pro-apoptotic genes increased. These studies further proved the correlation between age and chondrocyte apoptosis at the animal level and the phenomenon of apoptosis that existed in the early stage of OA ( Todd Allen et al., 2004 ). In another study involving horses, a positive correlation was found between the severity of chondrocyte injury and apoptosis. The expression level of pro-apoptotic factors is higher in horses that often suffer from OA. Apoptosis participates in the pathogenesis of OA. Apoptosis has been observed on human femoral head cartilage ( Thomas et al., 2011 ). In comparison with the average population, the expression of receptors and the degree of apoptosis in OA patients’ articular cartilage was much higher. In the late stages of OA, the degree of apoptosis become even worse ( Héraud et al., 2000 ).
Inflammatory Reaction
The inflammatory reaction is a basic pathological process mainly involving a defensive response when the body is stimulated. Inflammation is involved in the pathological process of many diseases, such as cancer ( Gianni et al., 2016 ), tendon or ligament injury ( Gracey et al., 2020 ). As early as the middle of the 19th century, studies pointed out that inflammation was closely related to OA. Articular chondrocytes and synovial cells all expressed inflammatory mediators ( Liu-Bryan, 2013 ). Inflammatory factors such as IL-1β and TNFα participate in the inflammatory response in articular chondrocytes and synovial cells ( Malfait, 2016 ). The cells mainly involved in the inflammatory response are macrophages and monocytes. The degree of inflammation is related to the degree of joint dysfunction and inflammatory factors ( Scanzello et al., 2011 ). In the early stage of OA, the expression of monocytes and inflammatory mediators increased, whereas with the continuous development of OA, the expression of monocytes and inflammatory mediators was gradually decreased ( Benito et al., 2005 ).
Rosshirt et al. (2019) used the knee joints of 55 OA patients as the research object, described the activation state of T cells, and found that a large proportion of T cells were activated to participate in OA inflammation, which mainly affected the joint itself the most. Inflammation can promote catabolism and participate in the pathogenesis of the disease. van den Bosch (2019) found a large number of inflammatory mediators in the tissues of patients with OA. These inflammatory mediators can induce the generation of degrading enzymes in articular cartilage, leading to the degradation of the ECM and the destruction of cartilage tissue. Lieberthal et al. (2015) established a post-traumatic OA model and observed the relationship between inflammation and OA progression. They found that an inflammatory reaction occurred in the early stage of OA. In patients with, multiple inflammatory pathways were activated and produced multiple inflammatory mediators such as cytokines and chemokines, which played an essential role in the pathogenesis of OA ( Scanzello, 2017a ). The genomic expression profiles of OA patients have been collected and subjected to meta-analysis, and the results show that MAP kinase, NF-κB activation, and oxidative phosphorylation can induce inflammatory signals and participate in the pathological change mechanism of OA ( Li et al., 2014 ). Macrophages are immune cells that play an essential role in the inflammatory response ( Bondeson et al., 2010 ). Woodell-May and Sommerfeld (2020) explored the role of immune cells such as macrophages in the synovial membrane of patients with OA. They found that in OA, macrophages could release oxygen free radicals, proteases, and inflammatory factors, thus affecting the microenvironment of inflammation. In the wound healing stage, macrophages also release IL-1, IL-6, and other inflammatory factors to regulate the inflammatory response. By stimulating protease, inflammation can cause cartilage degeneration and eventually induce OA. Based on the relationship between mechanical injury and OA, mechanical damage can lead to mechanical inflammation, which activates NF-kB and inflammatory mitogen-activated protein kinase, thereby causing a series of functional problems of the join ( Vincent, 2019 ). If the rash persists for a long time, it will affect the repair of cartilage tissue. Based on the summary of articles related to inflammation in OA ( Goldring and Otero, 2011 ), when some risk factors inducing OA appear, the expression of pro-inflammatory factors and various related enzymes are upregulated in articular cartilage and synovial tissue through specific signaling pathways. Inflammatory elements, which are essential for cartilage damage and repair, can change adjacent joint tissues and form a vicious circle ( Houard et al., 2013 ). The knee joint is damaged under severe mechanical stimulation, causing catabolism and stress response of chondrocytes, and finally inducing inflammation, leading to joint pain. Inflammation is one of the important mechanisms that lead to the pathological changes of joint cartilage and synovial membrane ( Scanzello, 2017b ).
During autophagy, cells degrade their damaged organelles and macromolecular substances by using lysosomes to regulate autophagy-related genes, which can achieve the renewal of organelles and is essential for cell metabolism ( Guo et al., 2021 ). Autophagy is involved in the occurrence of OA. Through cellular autophagy, the function of damaged articular cartilage can be restored, thereby alleviating the pathological process of OA. Excess ROS will lead to cartilage degradation and inhibit the synthesis of ECM. Kongara and Karantza (2012) showed that autophagy could maintain the typical morphology of cartilage and the dynamic balance of ECM synthesis and metabolism by regulating the body’s ROS.
Barranco (2015) found that the inhibition of the Akt-mTOR signaling pathway in chondrocytes can promote the autophagy of articular chondrocytes and regulate oxidative stress ( Xue et al., 2017 ), thus participating in the development process of OA. If autophagy is activated, the damaged mitochondria and peroxidase bodies are removed, thus inhibiting the production of reactive oxygen species in the body and protecting the articular cartilage from pathological changes. Based on the summary of the roles of autophagy in OA, in the early stage of OA, moderate autophagy contributes to the survival of chondrocytes and is essential for preventing and delaying OA ( Duan et al., 2020 ). Li et al. (2016) found that autophagy was mainly committed in the chondrocytes of patients with OA. By regulating oxidative stress response and apoptosis, the pathological changes of the knee joint can be alleviated, and chondrocytes can be protected from various stimulations. With the growth of age, the incidence of OA increases gradually, and this phenomenon is related to autophagy. Autophagy gradually weakens with age. Therefore, the structure and function of articular cartilage decreases with age, leading to pathological changes in the knee joint ( Mathew et al., 2009 ). Caramés et al. (2010) that in OA animal models, the expression of autophagy regulatory factors is downregulated, and the steady-state of cartilage is damaged, indicating that autophagy can protect articular cartilage and play an essential role in maintaining cartilage steady state. The decrease of autophagy will induce OA. Taking articular cartilage of OA and non-OA patients as the research object, we can find that autophagy regulates the expression of OA-related genes. In comparison with patients without OA, autophagy is expressed in the chondrocytes of OA patients at a higher level, and the manifestation of autophagy markers is also upregulated ( Sasaki et al., 2012 ). Similarly, Chang et al. (2013) took human chondrocytes as the research object and found that many autophagy-related proteins are expressed in the articular cartilage of patients with OA. In the pathogenesis of OA, autophagy plays a role in protecting chondrocytes and promoting metabolism. However, excessive autophagy causes a large number of chondrocyte deaths.
Changes in Non-coding RNA
Non-coding RNA (ncRNA) is an RNA that does not code for protein, mainly including miRNA, lncRNA, and circRNA. These RNAs can exert biological functions at the RNA level without being translated into proteins. ncRNA plays an essential role in the pathological process of many diseases, such as cardiovascular disease ( Jusic and Devaux, 2020 ), cancer ( Karreth and Pandolfi, 2013 ), diabetes ( Beltrami et al., 2015 ). ncRNA also plays a vital role in the process of OA. It can promote or inhibit cartilage formation by regulating the degradation of cell-matrix, cell proliferation, apoptosis, and inflammatory response, thereby inducing or treating OA ( Hong and Reddi, 2012 ).
Changes in the expression levels of many miRNAs can induce pathological changes in OA. MiR-204/-211 and miR-29b-3p are common miRNAs, which are differentially expressed in patients with OA. Huang et al. (2019) established an OA mouse model and found that miR-204/-211 was missing, and Runx2 was increased. Mesenchymal progenitor cells proliferated abnormally at the same time by Micro-CT and histological determination. Akt signal North is activated, thus inducing the dysfunction of various components in the joint and finally leading to OA. Therefore, miR-204/-211 can maintain intra-articular homeostasis and ensure that articular chondrocytes and synovial cells function usually. Chen et al. (2017) used rat chondrocytes as the research object. Through luciferase reporter gene detection, they found that miR-29b-3p in patients with OA was upregulated, and miR-29b-3p ultimately led to the degeneration of articular cartilage tissue by targeting and inhibiting the expression of rat GRN mRNA. In addition, microarray technology was used to detect the expression of miRNA in chondrocytes. They found that miRNA-140, miRNA-455, miR-146a, miR-155, and miR125b differed in OA patients and the average population, thus inducing pathological changes of articular cartilage, synovial membrane, and subchondral bone ( Swingler et al., 2012 ). lncRNA also plays a vital role in OA. Throughout the cartilage development, different lncRNA is regulated, and the regular expression of lncRNA can prevent cartilage differentiation disorders. During cartilage degeneration, other lncRNA plays various roles ( Zhu et al., 2019 ). Ye et al. (2018) studied the articular cartilage of patients with OA. They found that the expression of ZFAS1 in OA chondrocytes was downregulated, and ZFAS1 might reduce the activity of chondrocytes and induce pathological changes of articular cartilage tissues by targeting the Wnt3a signaling pathway. Similarly, the role of circa in OA has been widely studied. Cyclic RNA plays a multi-faceted regulatory role in the progression of OA ( Zhang W. et al., 2021 ). Chen et al. (2020) found that in OA tissues, the expression levels of circRNA-UBE2G1 and HIF-1a were significantly increased, while the expression of miR-373 was downregulated. Functional testing showed that circRNA-UBE2G1 could bind to miR-373, thereby inhibiting the interaction of miR-373 and HIF-1a, damaging the chondrocytes, and leading to a series of pathological changes. In conclusion, ncRNA is closely related to OA. The differential expression of miRNA, lncRNA and circRNA in healthy people and OA patients can lead to pathological changes in articular cartilage, synovial membrane, and subchondral bone and affect the pathological process of OA.
Methylation
Methylation is the catalyzed transfer of methyl groups from an active compound to another compound. This process can form various methyl compounds or result in chemical modification of particular proteins or nucleic acids to start methylation products ( Urnov, 2002 ). Methylation mainly includes DNA methylation and m6a methylation ( Dai et al., 2021 ).
DNA methylation is a chemical modification of DNA that alters genetic behavior without altering the DNA sequence. Its primary process is under the action of DNA methyltransferase. DNA methylation can cause changes in chromatin structure, DNA conformation, DNA stability, and the way DNA interacts with the protein, thereby controlling gene expression ( Reynard, 2017 ). When CtBP1 and CtBP2 are overexpressed, the pro-inflammatory factors are increased, while the NLRP3 signaling pathway is activated to induce OA. DNA methylation in the CtBPs promoter reduces the expression level of CtBPs in OA tissues and regulate CtBP-mediated signal transduction, finally participating in the pathogenesis of OA ( Sun et al., 2020 ). In addition, DNA methylation can participate in the pathogenesis of OA by affecting the expression of genes such as matrix metalloproteinase-3, matrix metalloproteinase-9, and type II collagen ( Cui and Xu, 2018 ).
N6- methyladenine (m6a) is one of the most abundant chemical modifications of eukaryotic messenger RNA. m6a modifications mainly include m6a methyltransferase catalysis, m6a demethylase removal, and m6a binding protein recognition ( Ma and Ji, 2020 ). This process is widely involved in regulating various life cycle stages such as mRNA splicing, processing, translation, and degradation. It is related to osteosarcoma, rheumatoid arthritis, osteoporosis, OA, and abnormal physiological functions ( Zhang W. et al., 2020 ). The strange expression of m6a-related gene and protein in OA can trigger the imbalance of m6a methylation, regulate the expression of OA-related genes to participate in the occurrence and development of OA, and is closely related to the poor prognosis of patients ( Yang et al., 2021 ). METTL3 is a methylated gene of m6a. He et al. (2021) studied mice in vivo and in vitro and induced the OA model with inflammatory stimuli such as TNF-α. The results showed that the expression of METTL3 was decreased in the OA model. Bcl2 is a downstream target gene of METTL3, and the presentation of METTL3 can promote m6A methylation of Bcl2 mRNA, thereby inhibiting chondrocyte apoptosis and autophagy. The detection of synovial tissue in patients with OA revealed that METTL3 could also induce autophagy by inhibiting ATG7 ( Chen X. et al., 2021 ). In addition, METTL3 may participate in OA by regulating the inflammatory response ( Sang et al., 2021 ). In summary, methylation is also an essential mechanism for pathological changes in OA. Furthermore, the epigenetic mechanism plays an essential role in the pathogenesis of OA.
Mechanism of Exercise Improving Osteoarthritis
Exercise is good medicine. An increasing number of studies supports that exercise can enhance physical fitness, build up the body, and prevent and treat certain diseases, playing an increasingly important role in people’s lives ( Ruegsegger and Booth, 2018 ). Studies ( Pedersen and Saltin, 2015 ) have summarized the mechanism of exercise in treating 26 different conditions, providing a theoretical basis for treating diseases by exercise. The treatment of OA by exercise has attracted increasing attention and has gradually become an OA research hotspot. In the present paper, the pathological mechanism of exercise in OA treatment was summarized through the induction of relevant literature, as shown in Table 1 . Exercise relieves the pathological changes of OA by affecting the degradation of the ECM, apoptosis, inflammatory response, autophagy, and changes of ncRNA. And training is used to treat OA ( Figure 2 ).
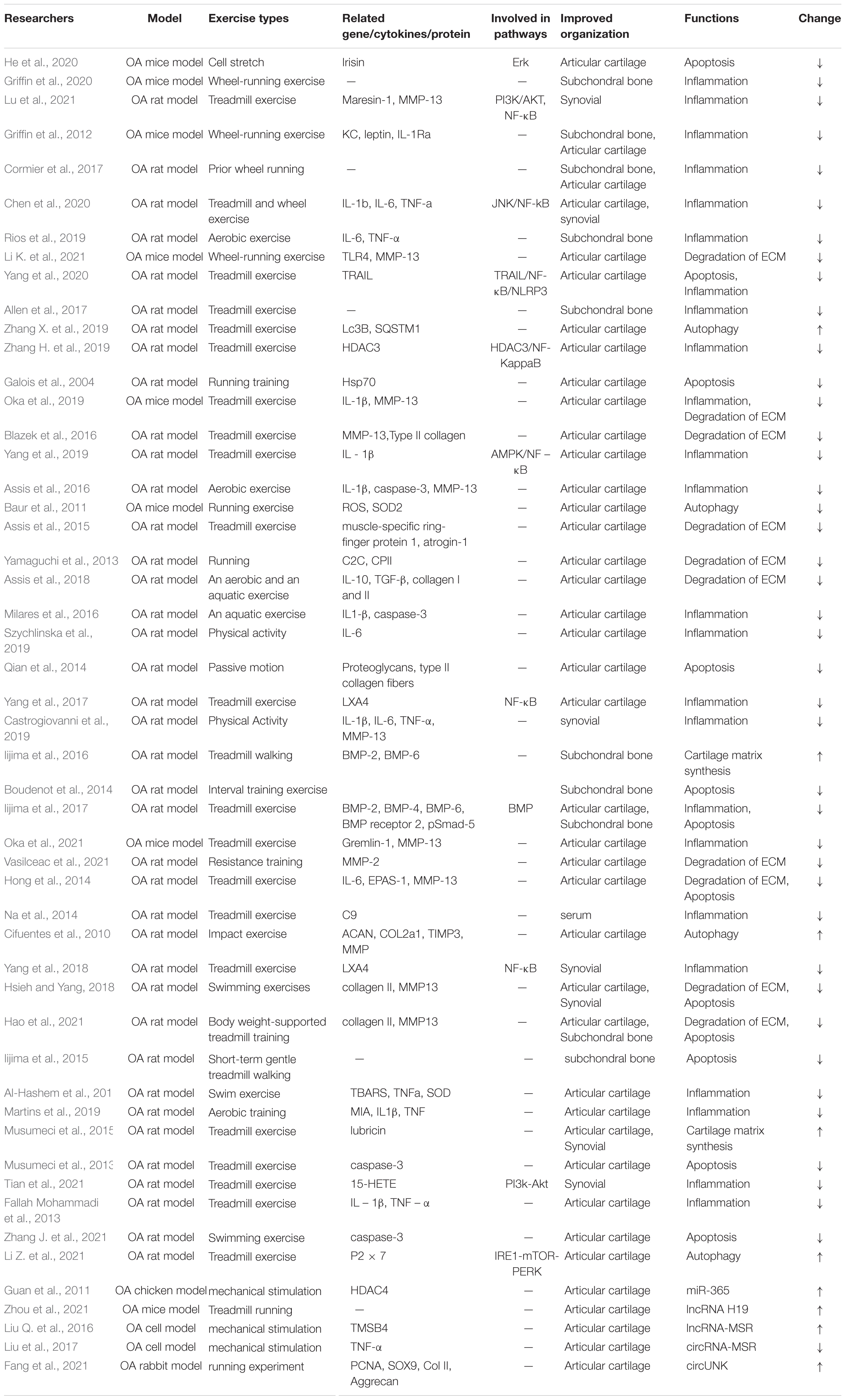
Table 1. Mechanism of exercise in the treatment of Osteoarthritis (OA).
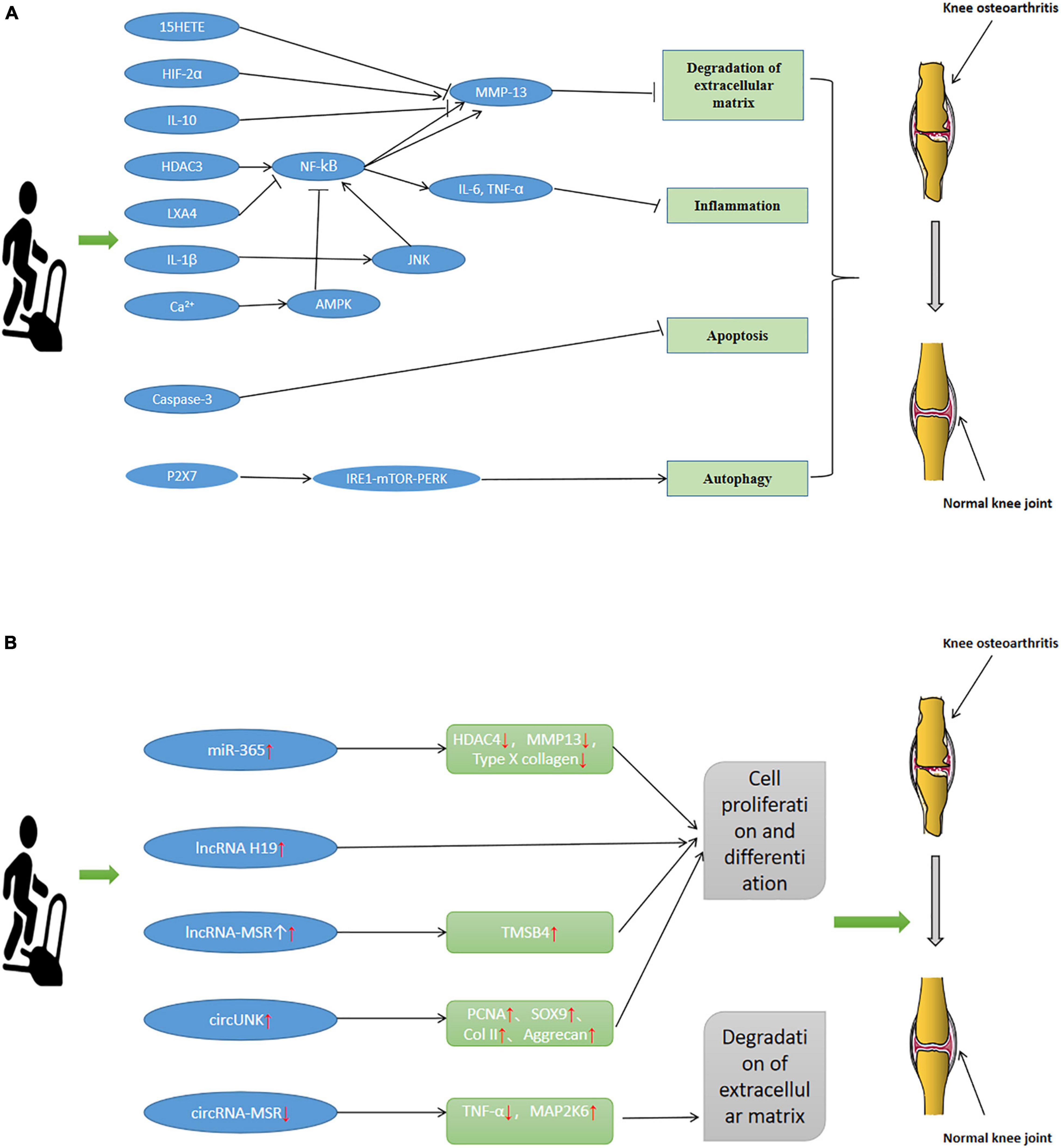
Figure 2. Pathological change mechanism of Osteoarthritis (OA). Exercise relieves the pathological changes of OA by affecting the degradation of the ECM, apoptosis, inflammatory response, autophagy and changes of ncRNA. (A) Mechanism of pathological changes of OA through degradation of ECM, apoptosis, inflammatory response, and autophagy. (B) Mechanism of pathological changes of OA through ncRNA.
The Role of Exercise in the Degradation of Extracellular Matrix
Exercise can delay the pathological process of OA by inhibiting the degradation of the ECM. Articular cartilage degradation is an essential pathological change of OA. Blazek et al. (2016) used OA rats as the research object. Exercise and non-exercise were used as intervention means to compare the whole gene expression of the transcriptome of articular cartilage in rats. Microarray analysis showed 644 differentially expressed genes in the articular cartilage of exercise rats. Therefore, exercise can prevent OA by changing genes related to OA’s pathogenesis and regulating the degradation and synthesis of ECM by changing metabolic pathways to alleviate the pathological changes of articular cartilage with sound prevention effects on OA. An OA rat model has been established to evaluate the expression of IL-10, TGF-β, and collagen type I and II in articular cartilage. The presentation of the above biochemical indicators increased in the articular cartilage of mice after exercise, indicating that exercise is very beneficial to articular cartilage. Moreover, considering that collagen type II is an essential component of ECM, exercise may delay the progression of OA by inhibiting the degradation of ECM and promoting cartilage synthesis ( Assis et al., 2018 ). Vasilceac et al. (2021) conducted resistance training for eight weeks for OA rats. They analyzed whether ELISA changed the activity of MMP-2 in the tendons around the knee joint and finally found that exercise could reduce the activity of MMP-2 in the quadriceps tendon of OA rats and greatly relieve the adverse effects of OA on the knee joint. Results show that exercise may reduce the degradation of the ECM by inhibiting the activity of MMP-2 from achieving the purpose of treating OA. Exercise in different stages of the course of OA produces other effects. Type II collagen (CoII) and matrix metalloproteinase-13 (MMP-13) are essential components related to the degradation of the ECM. Hsieh and Yang (2018) established an OA rat model, in which the samples were allowed to swim in different stages of the course of OA. They found that swimming training in the early stage of OA could increase CoII. The level of MMP-13 can be reduced to maintain a balance between the degradation and anabolism of ECM and prevent cartilage damage through exercise training in the early stage of OA rather than that in the late stage.
Overall, the degradation of the ECM is the pathological change mechanism of OA. Exercise can protect articular cartilage and delay the progression of OA by inhibiting this mechanism, and it is a crucial intervention to prevent and treat OA.
The Role of Exercise in Apoptosis
Apoptosis is one of the mechanisms for the pathological changes of OA. Exercise delays the pathological process of OA by inhibiting apoptosis. Taking articular cartilage of OA rats as a research sample, Yang et al. (2020) detected the expression of relevant genes in articular cartilage after exercise through bioinformatics analysis. Results show that the levels of TRAIL, NF-κB p65, and NLRP3 in cartilage after activity were decreased. Exercise could inhibit apoptosis and prevent OA by regulating the TRAIL/NF-κB/NLRP3 signaling pathway of OA. By comparing OA rats with mild, moderate, and high-intensity training and comparing the expression of caspase three and Hsp70, we found that mild and moderate exercise could inhibit apoptosis and protect the articular cartilage ( Galois et al., 2004 ). Qian et al. (2014) established an OA rat model, and the experimental group was subjected to passive motion. Based on the measurements of the proteoglycan content of the cartilage matrix, the number of type II collagen fibers, and apoptotic chondrocytes in the experimental and control group, the changes in biochemical signals caused by passive exercise in the early and middle stages of OA differed. In comparison with the control group, the cartilage matrix proteoglycan content and type II collagen fiber level remarkably increased three weeks after passive motion in the early stage of OA. The number of apoptotic cells was significantly reduced. However, three weeks after passive exercise in the middle stage of OA, the number of apoptotic cells was not significantly changed. Therefore, passive exercise at the early stage of OA may delay articular cartilage degeneration by inhibiting apoptosis, preventing, and treating OA. After intermittent training for OA rats, the pathological changes of their subchondral bones were assessed by immunolabeling with cleaved caspase-3 in the cortical subchondral bone. Intermittent aerobic training may prevent the OA-induced reduction in bone mineral density by reducing apoptosis ( Boudenot et al., 2014 ). Studies ( Iijima et al., 2015 ) have established the OA rat model and analyzed the pathological conditions of rat articular cartilage and subchondral bone before and after four weeks of exercise. They found that exercise might inhibit apoptosis and protect cartilage tissue, thus delaying OA progression. Musumeci et al. (2013) established the OA rat model to study the effect of exercise on articular cartilage. First, drugs were used to induce pathological changes in the articular cartilage. Then, exercise intervention was performed on rats to observe the morphological changes of articular cartilage. The results show that exercise might induce the expression of lubricating oil and inhibit the activity of caspase-3, thereby reducing apoptosis and delaying or even reversing pathological changes of articular cartilage. In some studies ( Zhang J. et al., 2021 ) concerning establishing the OA rat model, the experimental group was intervened by swimming for four weeks. The morphological changes of cartilage were analyzed by hematoxylin-eosin (H&E) staining. The expression of caspase-3 was analyzed by Western blot and qPCR. The results showed that after four weeks of swimming, the abnormal morphology of articular cartilage was improved, and the level of caspase-3 protein decreased, indicating that exercise might reduce apoptosis by inhibiting the level of caspase-3 protein, thereby protecting articular cartilage and preventing and treating OA.
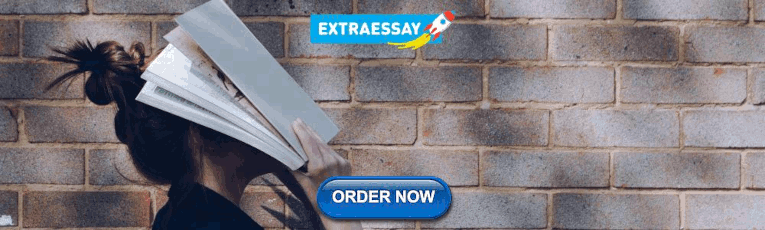
The Role of Exercise in Inflammatory Response
Exercise can inhibit the inflammatory response by reducing pro-inflammatory factors, thereby delaying the pathological changes of OA, which is the most common mechanism of activity in OA treatment. The inflammatory response mechanism of exercise therapy for OA has been widely studied, and many related pathways regulate the inflammatory response of OA, such as PI3k/Akt, NF-κB p65, JNK/NF-kB, HDAC3/NF-kappaB, and AMPK/NF-κB signaling pathways. Lu et al. (2021) established the OA rat model and allowed them to exercise on the treadmill. They found that after running exercise, the mice produced maresin-1, and the increased level of maresin-1 activated the PI3k/Akt pathway and inhibited the NF-κB p65 pathway, thus playing an anti-inflammatory role and delaying the pathological changes of OA. Griffin et al. (2012) established the OA mouse model by inducing a high-fat diet and then letting the mice run to observe the expression of inflammation-related factors. Finally, they found that exercise could regulate the expression of pro-inflammatory factors, promote joint health, and reduce the severity of pathological changes in joints of OA mice. To study the signal transduction of JNK/NF-kB in KOA patients, we established the 0A rat model and conducted a controlled intervention experiment. Finally, the knee joint diameter in the exercise group is lower than that in the OA group, indicating that exercise is conducive to KOA recovery. The IL-1b, IL-6, and TNF-a levels decreased, indicating that exercise can reduce the inflammatory response by regulating the JNK/NF-kB signaling pathway, which ultimately can delay the pathological changes of OA ( Chen et al., 2020 ). Osteoarthritis rats were subjected to moderate-intensity exercise on the treadmill. The H&E staining and toluidine blue O staining were used to detect cartilage injury. The expression levels of some biochemical signals in the articular cartilage were examined via immunohistochemistry and other methods. The results showed that moderate-intensity treadmill exercise could reduce the inflammatory response and protect the articular cartilage by inhibiting the HDAC3/NF-kappaB pathway ( Zhang H. et al., 2019 ). Similarly, Yang et al. (2019) intervened OA rats with treadmill exercise and used the articular cartilage as the research object. Observation and analysis results show that moderate-intensity exercise can reduce the sensitivity of articular cartilage and chondrocytes to inflammatory response through the AMPK/NF-κB signaling pathway, and it is an essential mechanism for reversing the pathological changes of articular cartilage. The analysis of biochemical indicators of OA rats after aerobic exercise show that the expression levels of IL-1β, caspase-3, and MMP-13 in OA rats decreased after exercise, and aerobic exercise could inhibit the inflammatory response of OA and prevent and treat the pathological changes of cartilage ( Assis et al., 2016 ). After water sports, OA rats’ articular cartilage and inflammatory mediators were changed. After eight weeks of the experiment, the cell damage degree of the rat was relieved, and the expression levels of IL-1β and caspase-3 were reduced. Therefore, sports can regulate OA inflammatory factors and inhibit inflammatory reactions to treat OA ( Milares et al., 2016 ).
The Role of Exercise in Autophagy
In OA, autophagy is a cellular protective response. Exercise can protect the knee joints of KOA patients by inducing autophagy, which is mainly regulated by oxidative stress. Relatively few studies have demonstrated the specific mechanism pathways through which exercise protects the knee joint through autophagy. Zhang X. et al. (2019) used sodium iodoacetate to induce OA rat model, and the experimental group was subjected to treadmill exercise for four weeks. The study found that serum IL-1β was decreased, IL-4 was increased, and the expression of type II collagen in articular cartilage was increased in rats undergoing treadmill exercise compared with those in OA rats that did not experience any activity. Therefore, treadmill exercise may protect the knee joints of OA patients by promoting the autophagy of articular cartilage. Baur et al. (2011) established an OA mouse model to study the effect of running on articular cartilage and bone of OA patients and observed the changes of biochemical signals and articular cartilage of running mice after eight weeks. They found that the content of reactive oxygen species in mice increased after exercise. Exercise can promote autophagy and protect the articular cartilage and bone of OA patients by regulating oxidative stress. Another study ( Cifuentes et al., 2010 ) has established an OA rat model. The experimental group was exposed to treadmill exercise for 8 weeks to observe the pathological changes of articular cartilage. In comparison with OA rats that did not undergo exercise, the protein and polysaccharide contents on the surface of the articular cartilage of rats after eight weeks of the exercise was relatively high. This experiment confirmed that training can enhance the oxidative stress mechanism and protect articular cartilage. Moderate-intensity exercise plays different roles in different stages of OA progression. In the early stage of OA, the average power of movement can delay the progression of OA by promoting autophagy. In the late stage of OA, moderate-intensity exercise can over-activate purinergic receptor P2X ligand-gated ion channel 7 and increase the number of apoptotic cells, thus aggravating the progression of OA. Throughout the OA progression, the body may promote autophagy or apoptosis through the IRE1-mTOR-PERK signal axis to delay or accelerate the pathological process of OA ( Li Z. et al., 2021 ).
The Role of Exercise in Non-coding RNA
Exercise can alleviate the pathological changes of OA by regulating the expression of ncRNA. The practice affect the face of miRNA, lncRNA, and circRNA in articular cartilage, synovial membrane, and subchondral bone of OA patients.
miRNA is related to maintaining the typical morphology of articular cartilage. The detection of miRNA expression in OA rats revealed that compared with the non-exercise rats, 394 differentially expressed miRNAs were detected in the exercise rats ( Yang et al., 2020 ). The analysis of articular cartilage from different animals revealed that exercise could change the miRNA expression and improve the pathological changes of articular cartilage through a series of mechanisms. Dunn et al. (2009) analyzed the expression of miRNA in bovine articular cartilage. They found that the expression patterns of miR-221 and miR-222 in weight-bearing medial anterior condylar articular cartilage was higher than those in non-weight-bearing medial posterior condylar articular cartilage. When the articular cartilage was loaded with weight, miR-221 downregulated type II collagen and Sox9, and miR-222 downregulated HDAC4 and MMP-13, maintaining the typical morphology of articular cartilage. Guan et al. (2011) subjected chicken chondrocyte samples to mechanical stimulation to analyze the roles of relevant miRNA in the mechanical stimulation using microarray technology. Results show that mechanical stimulation could upregulate the miR-365 expression in chondrocytes. miR-365 stimulated the differentiation of chondrocytes by targeting histone deacetylase 4 (HDAC4) and finally alleviated the pathological process of cartilage tissue. In addition, the expression levels of many lncRNA changed during exercise. Zhou et al. (2021) established an OA mouse model. Mice with moderate-intensity exercise intervention had an upregulated lncRNA H19 expression, thickened articular cartilage, and resolved OA compared with mice without exercise intervention. Under the stimulation of mechanical stress, lncRNA-MSR was activated and then competitively bound to miR-152, thereby promoting the expression of TMSB4 and leading to the degradation of the ECM ( Liu Q. et al., 2016 ). The regulatory role of circRNA during exercise is also crucial. A total of 104 circRNA were differentially expressed (44 upregulated and 60 downregulated), with increased circRNA-MSR expression, in damaged articular cartilage compared with intact articular cartilage. circRNA-MSR expression was downregulated after mechanical stress was applied to the cartilage. The downregulation of circRNA-MSR can inhibit the expression of TNF-α and promote the degradation of the extracellular chondrocyte matrix, thus enhancing the structure and function of cartilage ( Liu et al., 2017 ). In addition, circRNA-MSR can directly target miR-643 to u-regulate MAP2K6. The downregulation of circRNA-MSR expression can promote cell proliferation and reduce cartilage damage ( Jia and Wei, 2021 ). qRT-PCR and Western blot have been used to analyze the genes related to cartilage differentiation in cartilage tissues. The results show that the expression of circUNK was upregulated, which improved the cartilage injury in OA rabbit and promoted the expression of molecules related to cartilage differentiation, such as PCNA, SOX9, Col II, Aggrecan, and protein-polysaccharide. These molecules had sound effects on the proliferation and differentiation of articular cartilage. In conclusion, exercise or mechanical stimulation can lead to changes in the expression of ncRNA, which prevents and improves the pathological changes of articular cartilage, synovial membrane, and subchondral bone through a series of mechanisms, thereby delaying the progression of OA ( Fang et al., 2021 ).
Effects of Different Exercise Types on Human Osteoarthritis
Many types of training can relieve pain, enhance muscle strength and improve joint stiffness ( Daenen et al., 2015 ). For healthy people, exercise can promote good health, while for OA patients, dysfunction can be improved and diseases can be treated ( Skou et al., 2018 ). At present, there are many types of sports for the treatment of OA, such as aerobic exercise, anti-resistance exercise, neuromuscular training, etc ( Jansen et al., 2011 ). In addition, Chinese traditional sports such as Baduanjin, Wuqinxi, and yoga are also applied to the prevention and treatment of OA ( Li et al., 2020 ). This paper summarizes the research on training types of OA patients by summarizing related literature, as shown in Table 2 .
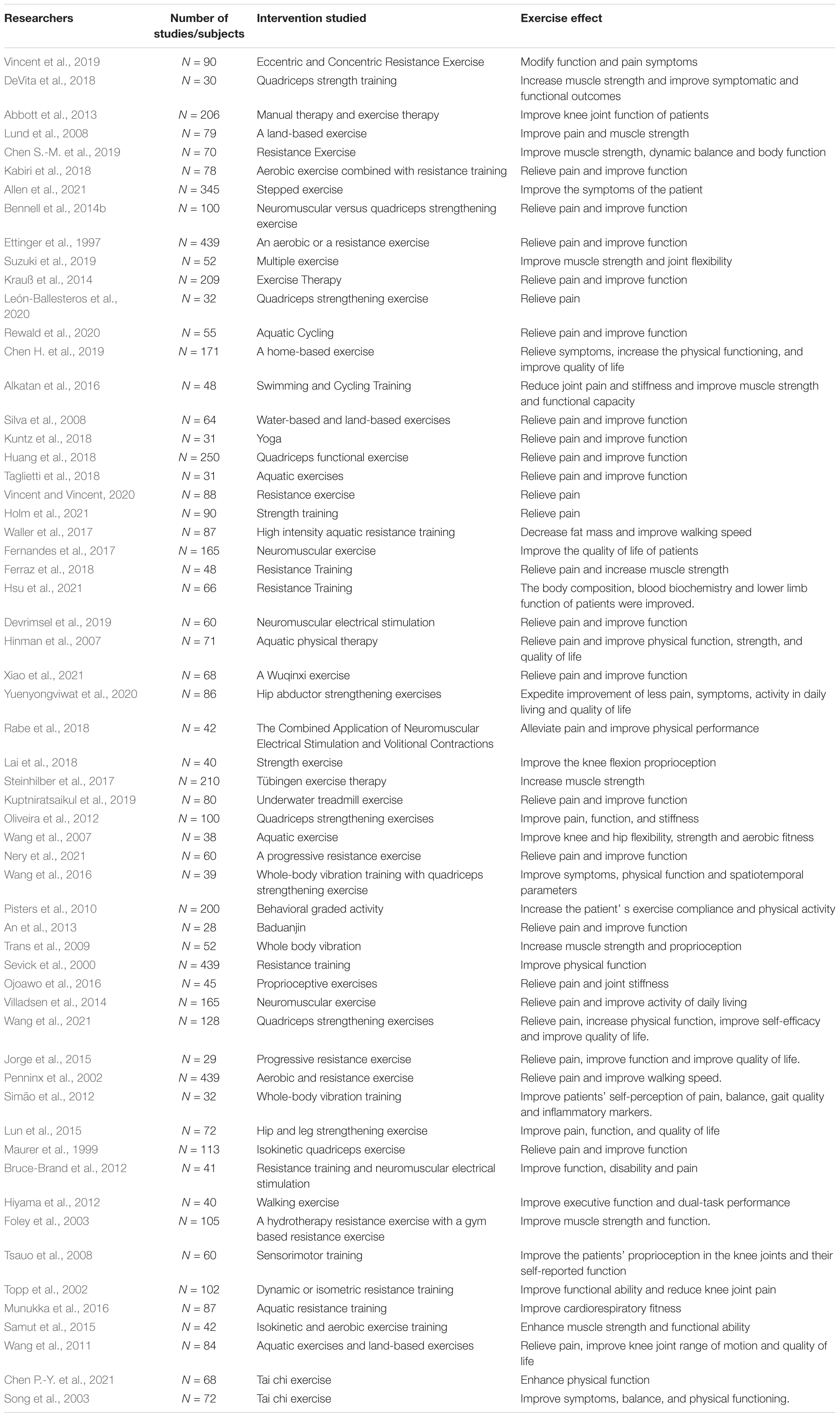
Table 2. Different exercise types on human Osteoarthritis (OA).
Conclusion and Outlook
At present, the incidence of OA is very high, and its pathogenesis remains unclear. The treatment of OA by exercise has received increasing research attention. Not all types of sports used can alleviate OA. The result of different types, intensity, and time of sports on OA differs.
Some studies believe that exercise does not affect OA. For example, Rios et al. (2020) intervened in KOA rats with aerobic exercise for 12 weeks and measured bone mineral density and knee joint injury. The results showed that training did not affect the common knee injury, accelerate the progression of OA, and alleviate the pathological changes of OA. Other studies believe that exercise accelerates the progression of OA. For example, Liu S.-S. et al. (2016) established a rat model of exercise-induced OA. They analyzed and compared the expression levels of various related mRNA, protein, and pathways in the standard and injury group of exercise-induced OA by using RT-qPCR, Western blot analysis, and immunohistochemical staining. The results showed that excessive pressure would lead to abnormal expression of many biochemical signals and abnormal activation of the Wnt/β-catenin pathway, leading to induced OA. Exercise has different effects on healthy and damaged articular cartilage ( Rojas-Ortega et al., 2015 ). Notably, for articular cartilage injury, if high-intensity practice is continued, the activity of catabolic proteins is more vital than that of anabolic proteins. The body’s metabolism is imbalanced, thus efficiently inducing OA. Twelve weeks of high-intensity treadmill training in rats will increase the levels of some apoptosis-related proteins and inflammation-related factors, such as caspase-3, IL-1α, and TNF-α, which is the precursor of OA ( Franciozi et al., 2013 ). The results indicate that high-intensity exercise may lead to the occurrence and development of OA. In addition, moderate exercise can delay the pathological process of OA. For example, a daily external force has been used to compress OA mice under low or medium intensity, and the pathological changes of articular cartilage were observed in the second and sixth weeks. Moreover, low- and medium-intensity compression can reduce the degeneration of articular cartilage and osteophytes of subchondral bone in mice and is very beneficial to the damaged joints, making it an effective method for the treatment of OA ( Holyoak et al., 2019 ).
At present, many exercises have therapeutic effects on OA, such as aerobic exercise, strength training, swimming, neuromuscular exercise, proprioceptive training, and balance training. Different types of motion produce other effects. Aerobic exercise is the most widely used in OA patients, and it can reduce the expression of IL-1β, caspase-3 and MMP-13 and prevent cartilage degradation in KOA rats ( Assis et al., 2016 ). Aerobic exercise may be the best training method to reduce pain and improve body function ( Goh et al., 2019 ). However, aerobic exercise with different intensities has different effects on OA patients with varying degrees of injury. Low-intensity aerobic exercise had a better therapeutic effect in patients with severe OA ( Messier et al., 2021 ), while high-intensity aerobic exercise had a better therapeutic effect in patients with mild OA ( Multanen et al., 2017 ). In addition, strength training could reduce the activity of MMP-2 in the quadriceps tendon of the OA rat model ( Vasilceac et al., 2021 ), which was the most effective in improving muscle strength, and neuromuscular training was the best training method to relieve OA pain ( Ageberg and Roos, 2015 ). Swimming intervention in the early stage of OA can alleviate the stiffness of the knee joint and is better than land exercise ( Lund et al., 2008 ; Hsieh and Yang, 2018 ; Munukka et al., 2020 ). Land exercise can be performed after the patients’ joints have a certain degree of flexibility. Land exercise is better than water exercise in improving pain and enhancing function ( Escalante et al., 2011 ). Many traditional techniques are being applied more and more in OA, such as Baduanjin ( An et al., 2008 ), tai chi chuan ( Zhang Z. et al., 2020 ), Wuqinxi ( Xiao and Li, 2021 ), and yoga ( Kuntz et al., 2018 ). They can improve the physical function of OA patients and have a significant impact on the psychological status of OA patients. In addition, new intervention methods such as virtual reality and sports games have been gradually used to improve people’s physical and psychological conditions ( Sadeghi and Jehu, 2022 ). These sports have excellent development prospects in the treatment of OA.
Although many types of exercise can be used to prevent or treat OA, our most recommended method is to formulate a unique exercise prescription for each patient with OA based on the FITT (Frequency, Intensity, Time, and Type) principle. According to the exercise prescription, a study ( de Rooij et al., 2017 ) formulated a personalized rehabilitation program for 126 OA patients for 20 weeks. It was finally found that personalized exercise therapy could effectively improve the OA of the knee joint and the body function of the patients. Exercise prescription recommendations for patients with OA are as follows ( Bennell et al., 2014a ): (1) Exercise frequency: It is recommended that in the initial stage, the patient had better exercise at least 12 times within three months to master the skills and ensure compliance, and then the frequency was gradually increased and maintained to two to three times a week, and a week of moderate-intensity exercise lasting 150 to 300 min was accumulated; (2) Exercise intensity: Moderate intensity exercise is recommended. Namely, the heart rate is 120–150 beats/min, and the oxygen consumption during exercise is 50–70% of the maximum oxygen consumption; (3) Exercise time: The recommended exercise time is 30–60 min per day. The exercise time was gradually increased from a short time to 30–60 min/d; (4) Exercise type: generally, aerobic exercise and strength training are recommended. Aerobic exercise such as swimming, Tai Ji Chuan, unfavorable for mountaineering, climbing stairs and other excessive weight-bearing movements, strength training have equal length, such as Zhang or isokinetic resistance movement, etc., recommend the weight-bearing exercise is given priority to; (5) Precautions: Pain relief can be obtained only after 8–11 weeks of exercise therapy. Continuous exercise for more than 12 weeks is generally recommended to relieve decreased muscle strength and muscle atrophy associated with OA. Patients should also receive regular education during training to improve their self-management awareness, compliance and exercise efficacy. It is normal to feel some discomfort or pain during exercise. Ice application is required at the joints for 15–20 min after training. If severe pain occurs during exercise or swelling aggravates the next day, you should timely adjust the amount of activity. Different types of patients may have other exercise prescriptions. Patients with higher obesity are more suitable for aquatic exercise. For patients with upper limb OA, emphasis should be placed on improving the affected joints’ range of motion and flexibility. For patients with lower limb OA, emphasis should be placed on improving patients’ muscle strength and body stability. Therefore, we should formulate individualized exercise prescriptions according to the degree of lesion and needs of OA patients.
This review describes the pathological change mechanism of OA and the molecular mechanism of exercise in the treatment of OA. By using RT-qPCR, Western blot analysis, and immunohistochemical staining, we have found that the expression levels of related mRNA, protein, and pathways were changed in OA patients after exercise. This review also summarized animal experiments related to exercise and OA and the role of different exercise types in human OA, described the research progress of exercise in the prevention and treatment of OA, and provided a basis for exercise intervention to treat OA in the future. Exercise, as an intervention means, has a great development prospect in the treatment of OA.
Author Contributions
X-AZ and X-QW: conceptualization, project administration, and funding acquisition. HK, X-AZ, and X-QW: writing – review and editing. All authors contributed to the article and approved the submitted version.
This study was supported by the Innovative Talents Support Program for Universities of Liaoning Province, No. WR2019024 and Shanghai Frontiers Science Research Base of Exercise and Metabolic Health.
Conflict of Interest
The authors declare that the research was conducted in the absence of any commercial or financial relationships that could be construed as a potential conflict of interest.
Publisher’s Note
All claims expressed in this article are solely those of the authors and do not necessarily represent those of their affiliated organizations, or those of the publisher, the editors and the reviewers. Any product that may be evaluated in this article, or claim that may be made by its manufacturer, is not guaranteed or endorsed by the publisher.
Abbott, J. H., Robertson, M. C., Chapple, C., Pinto, D., Wright, A. A., Leon de la Barra, S., et al. (2013). Manual therapy, exercise therapy, or both, in addition to usual care, for osteoarthritis of the hip or knee: a randomized controlled trial. 1: clinical effectiveness. Osteoarthritis Cartilage 21, 525–534. doi: 10.1016/j.joca.2012.12.014
PubMed Abstract | CrossRef Full Text | Google Scholar
Ageberg, E., and Roos, E. M. (2015). Neuromuscular exercise as treatment of degenerative knee disease. Exerc. Sport Sci. Rev. 43, 14–22. doi: 10.1249/JES.0000000000000030
Aigner, T., Kim, H. A., and Roach, H. I. (2004). Apoptosis in osteoarthritis. Rheum. Dis. Clin. North Am. 30, 639–653.
Google Scholar
Al-Hashem, F., El Karib, A. O., Bin-Jaliah, I., Dallak, M., Sakr, H. F., Eid, R. A., et al. (2017). Exercise protects against insulin-dependent diabetes-induced osteoarthritis in rats: a scanning electron microscopy study. Ultrastruct. Pathol. 41, 252–257. doi: 10.1080/01913123.2017.1313346
Alkatan, M., Baker, J. R., Machin, D. R., Park, W., Akkari, A. S., Pasha, E. P., et al. (2016). Improved function and reduced pain after swimming and cycling training in patients with osteoarthritis. J. Rheumatol. 43, 666–672. doi: 10.3899/jrheum.151110
Allen, J., Imbert, I., Havelin, J., Henderson, T., Stevenson, G., Liaw, L., et al. (2017). Effects of treadmill exercise on advanced osteoarthritis pain in rats. Arthritis Rheumatol. 69, 1407–1417. doi: 10.1002/art.40101
Allen, K. D., Woolson, S., Hoenig, H. M., Bongiorni, D., Byrd, J., Caves, K., et al. (2021). Stepped exercise program for patients with knee osteoarthritis: a randomized controlled trial. Ann. Intern. Med. 174, 298–307. doi: 10.7326/M20-4447
An, B., Dai, K., Zhu, Z., Wang, Y., Hao, Y., Tang, T., et al. (2008). Baduanjin alleviates the symptoms of knee osteoarthritis. J. Altern. Complement. Med. 14, 167–174. doi: 10.1089/acm.2007.0600
An, B.-C., Wang, Y., Jiang, X., Lu, H.-S., Fang, Z.-Y., Wang, Y., et al. (2013). Effects of Baduanjin () exercise on knee osteoarthritis: a one-year study. Chin. J. Integr. Med. 19, 143–148. doi: 10.1007/s11655-012-1211-y
Assis, L., Almeida, T., Milares, L. P., dos Passos, N., Araújo, B., Bublitz, C., et al. (2015). Musculoskeletal atrophy in an experimental model of knee osteoarthritis: the effects of exercise training and low-level laser therapy. Am. J. Phys. Med. Rehabil. 94, 609–616. doi: 10.1097/PHM.0000000000000219
Assis, L., Milares, L. P., Almeida, T., Tim, C., Magri, A., Fernandes, K. R., et al. (2016). Aerobic exercise training and low-level laser therapy modulate inflammatory response and degenerative process in an experimental model of knee osteoarthritis in rats. Osteoarthritis Cartilage 24, 169–177. doi: 10.1016/j.joca.2015.07.020
Assis, L., Tim, C., Magri, A., Fernandes, K. R., Vassão, P. G., and Renno, A. C. M. (2018). Interleukin-10 and collagen type II immunoexpression are modulated by photobiomodulation associated to aerobic and aquatic exercises in an experimental model of osteoarthritis. Lasers Med. Sci. 33, 1875–1882. doi: 10.1007/s10103-018-2541-6
Ayral, X., Pickering, E. H., Woodworth, T. G., Mackillop, N., and Dougados, M. (2005). Synovitis: a potential predictive factor of structural progression of medial tibiofemoral knee osteoarthritis – results of a 1 year longitudinal arthroscopic study in 422 patients. Osteoarthritis Cartilage 13, 361–367.
Barranco, C. (2015). Osteoarthritis: activate autophagy to prevent cartilage degeneration? Nat. Rev. Rheumatol. 11:127. doi: 10.1038/nrrheum.2015.12
Bauer, D. C., Hunter, D. J., Abramson, S. B., Attur, M., Corr, M., Felson, D., et al. (2006). Classification of osteoarthritis biomarkers: a proposed approach. Osteoarthritis Cartilage 14, 723–727.
Baur, A., Henkel, J., Bloch, W., Treiber, N., Scharffetter-Kochanek, K., Brüggemann, G.-P., et al. (2011). Effect of exercise on bone and articular cartilage in heterozygous manganese superoxide dismutase (SOD2) deficient mice. Free Radic. Res. 45, 550–558. doi: 10.3109/10715762.2011.555483
Belavy, D. L., Van Oosterwijck, J., Clarkson, M., Dhondt, E., Mundell, N. L., Miller, C. T., et al. (2021). Pain sensitivity is reduced by exercise training: evidence from a systematic review and meta-analysis. Neurosci. Biobehav. Rev. 120, 100–108. doi: 10.1016/j.neubiorev.2020.11.012
Beltrami, C., Angelini, T. G., and Emanueli, C. (2015). Noncoding RNAs in diabetes vascular complications. J. Mol. Cell. Cardiol. 89(Pt A), 42–50. doi: 10.1016/j.yjmcc.2014.12.014
Benedetti, M. G., Furlini, G., Zati, A., and Letizia Mauro, G. (2018). The effectiveness of physical exercise on bone density in osteoporotic patients. Biomed Res. Int. 2018:4840531. doi: 10.1155/2018/4840531
Benito, M. J., Veale, D. J., FitzGerald, O., van den Berg, W. B., and Bresnihan, B. (2005). Synovial tissue inflammation in early and late osteoarthritis. Ann. Rheum. Dis. 64, 1263–1267.
Bennell, K. L., Dobson, F., and Hinman, R. S. (2014a). Exercise in osteoarthritis: moving from prescription to adherence. Best Pract. Res. Clin. Rheumatol. 28, 93–117. doi: 10.1016/j.berh.2014.01.009
Bennell, K. L., Kyriakides, M., Metcalf, B., Egerton, T., Wrigley, T. V., Hodges, P. W., et al. (2014b). Neuromuscular versus quadriceps strengthening exercise in patients with medial knee osteoarthritis and varus malalignment: a randomized controlled trial. Arthritis Rheumatol. 66, 950–959. doi: 10.1002/art.38317
Bennell, K. L., Hunter, D. J., and Hinman, R. S. (2012). Management of osteoarthritis of the knee. BMJ 345:e4934. doi: 10.1136/bmj.e4934
Blazek, A. D., Nam, J., Gupta, R., Pradhan, M., Perera, P., Weisleder, N. L., et al. (2016). Exercise- driven metabolic pathways in healthy cartilage. Osteoarthritis Cartilage 24, 1210–1222. doi: 10.1016/j.joca.2016.02.004
Bondeson, J., Blom, A. B., Wainwright, S., Hughes, C., Caterson, B., and van den Berg, W. B. (2010). The role of synovial macrophages and macrophage-produced mediators in driving inflammatory and destructive responses in osteoarthritis. Arthritis Rheum. 62, 647–657. doi: 10.1002/art.27290
Boudenot, A., Presle, N., Uzbekov, R., Toumi, H., Pallu, S., and Lespessailles, E. (2014). Effect of interval-training exercise on subchondral bone in a chemically-induced osteoarthritis model. Osteoarthritis Cartilage 22, 1176–1185. doi: 10.1016/j.joca.2014.05.020
Brandt, K. D., Braunstein, E. M., Visco, D. M., O’Connor, B., Heck, D., and Albrecht, M. (1991). Anterior (cranial) cruciate ligament transection in the dog: a bona fide model of osteoarthritis, not merely of cartilage injury and repair. J. Rheumatol. 18, 436–446.
Braun, H. J., and Gold, G. E. (2012). Diagnosis of osteoarthritis: imaging. Bone 51, 278–288. doi: 10.1016/j.bone.2011.11.019
Bruce-Brand, R. A., Walls, R. J., Ong, J. C., Emerson, B. S., O’Byrne, J. M., and Moyna, N. M. (2012). Effects of home-based resistance training and neuromuscular electrical stimulation in knee osteoarthritis: a randomized controlled trial. BMC Musculoskelet. Disord. 13:118. doi: 10.1186/1471-2474-13-118
Burr, D. B., and Gallant, M. A. (2012). Bone remodelling in osteoarthritis. Nat. Rev. Rheumatol. 8, 665–673. doi: 10.1038/nrrheum.2012.130
Caramés, B., Taniguchi, N., Otsuki, S., Blanco, F. J., and Lotz, M. (2010). Autophagy is a protective mechanism in normal cartilage, and its aging-related loss is linked with cell death and osteoarthritis. Arthritis Rheum. 62, 791–801. doi: 10.1002/art.27305
Castrogiovanni, P., Di Rosa, M., Ravalli, S., Castorina, A., Guglielmino, C., Imbesi, R., et al. (2019). Moderate physical activity as a prevention method for knee osteoarthritis and the role of Synoviocytes as Biological Key. Int. J. Mol. Sci. 20:511. doi: 10.3390/ijms20030511
Chang, J., Wang, W., Zhang, H., Hu, Y., Wang, M., and Yin, Z. (2013). The dual role of autophagy in chondrocyte responses in the pathogenesis of articular cartilage degeneration in osteoarthritis. Int. J. Mol. Med. 32, 1311–1318. doi: 10.3892/ijmm.2013.1520
Chen, H., Zheng, X., Huang, H., Liu, C., Wan, Q., and Shang, S. (2019). The effects of a home-based exercise intervention on elderly patients with knee osteoarthritis: a quasi-experimental study. BMC Musculoskelet. Disord. 20:160. doi: 10.1186/s12891-019-2521-4
Chen, S.-M., Shen, F.-C., Chen, J.-F., Chang, W.-D., and Chang, N.-J. (2019). Effects of resistance exercise on glycated hemoglobin and functional performance in older patients with comorbid diabetes mellitus and knee osteoarthritis: a randomized trial. Int. J. Environ. Res. Public Health 17:224. doi: 10.3390/ijerph17010224
Chen, L., Li, Q., Wang, J., Jin, S., Zheng, H., Lin, J., et al. (2017). MiR-29b-3p promotes chondrocyte apoptosis and facilitates the occurrence and development of osteoarthritis by targeting PGRN. J. Cell. Mol. Med. 21, 3347–3359. doi: 10.1111/jcmm.13237
Chen, L., Lou, Y., Pan, Z., Cao, X., Zhang, L., Zhu, C., et al. (2020). Treadmill and wheel exercise protect against JNK/NF-κB induced inflammation in experimental models of knee osteoarthritis. Biochem. Biophys. Res. Commun. 523, 117–122. doi: 10.1016/j.bbrc.2019.12.014
Chen, X., Gong, W., Shao, X., Shi, T., Zhang, L., Dong, J., et al. (2021). METTL3-mediated mA modification of ATG7 regulates autophagy-GATA4 axis to promote cellular senescence and osteoarthritis progression. Ann. Rheum. Dis. 81, 87–99. doi: 10.1136/annrheumdis-2021-221091
Chen, P.-Y., Song, C.-Y., Yen, H.-Y., Lin, P.-C., Chen, S.-R., Lu, L.-H., et al. (2021). Impacts of tai chi exercise on functional fitness in community-dwelling older adults with mild degenerative knee osteoarthritis: a randomized controlled clinical trial. BMC Geriatr. 21:449. doi: 10.1186/s12877-021-02390-9
Cifuentes, D. J., Rocha, L. G., Silva, L. A., Brito, A. C., Rueff-Barroso, C. R., Porto, L. C., et al. (2010). Decrease in oxidative stress and histological changes induced by physical exercise calibrated in rats with osteoarthritis induced by monosodium iodoacetate. Osteoarthritis Cartilage 18, 1088–1095. doi: 10.1016/j.joca.2010.04.004
Cormier, J., Cone, K., Lanpher, J., Kinens, A., Henderson, T., Liaw, L., et al. (2017). Exercise reverses pain-related weight asymmetry and differentially modulates trabecular bone microarchitecture in a rat model of osteoarthritis. Life Sci. 180, 51–59. doi: 10.1016/j.lfs.2017.05.011
Cui, D., and Xu, X. (2018). DNA Methyltransferases, DNA methylation, and age-associated cognitive function. Int. J. Mol. Sci. 19:1315. doi: 10.3390/ijms19051315
Da, R.-R., Qin, Y., Baeten, D., and Zhang, Y. (2007). B cell clonal expansion and somatic hypermutation of Ig variable heavy chain genes in the synovial membrane of patients with osteoarthritis. J. Immunol. 178, 557–565.
Daenen, L., Varkey, E., Kellmann, M., and Nijs, J. (2015). Exercise, not to exercise, or how to exercise in patients with chronic pain? Applying science to practice. Clin. J. Pain 31, 108–114. doi: 10.1097/AJP.0000000000000099
Dai, X., Ren, T., Zhang, Y., and Nan, N. (2021). Methylation multiplicity and its clinical values in cancer. Expert Rev. Mol. Med. 23:e2. doi: 10.1017/erm.2021.4
de Rooij, M., van der Leeden, M., Cheung, J., van der Esch, M., Häkkinen, A., Haverkamp, D., et al. (2017). Efficacy of tailored exercise therapy on physical functioning in patients with knee osteoarthritis and comorbidity: a randomized controlled trial. Arthritis Care Res. 69, 807–816. doi: 10.1002/acr.23013
DeVita, P., Aaboe, J., Bartholdy, C., Leonardis, J. M., Bliddal, H., and Henriksen, M. (2018). Quadriceps-strengthening exercise and quadriceps and knee biomechanics during walking in knee osteoarthritis: a two-centre randomized controlled trial. Clin. Biomech. 59, 199–206. doi: 10.1016/j.clinbiomech.2018.09.016
Devrimsel, G., Metin, Y., and Serdaroglu Beyazal, M. (2019). Short-term effects of neuromuscular electrical stimulation and ultrasound therapies on muscle architecture and functional capacity in knee osteoarthritis: a randomized study. Clin. Rehabil. 33, 418–427. doi: 10.1177/0269215518817807
Duan, R., Xie, H., and Liu, Z.-Z. (2020). The role of autophagy in osteoarthritis. Front. Cell Dev. Biol. 8:608388. doi: 10.3389/fcell.2020.608388
Dunn, W., DuRaine, G., and Reddi, A. H. (2009). Profiling microRNA expression in bovine articular cartilage and implications for mechanotransduction. Arthritis Rheum. 60, 2333–2339. doi: 10.1002/art.24678
Elmore, S. (2007). Apoptosis: a review of programmed cell death. Toxicol. Pathol. 35, 495–516.
Escalante, Y., García-Hermoso, A., and Saavedra, J. M. (2011). Effects of exercise on functional aerobic capacity in lower limb osteoarthritis: a systematic review. J. Sci. Med. Sport 14, 190–198. doi: 10.1016/j.jsams.2010.10.004
Ettinger, W. H., Burns, R., Messier, S. P., Applegate, W., Rejeski, W. J., Morgan, T., et al. (1997). A randomized trial comparing aerobic exercise and resistance exercise with a health education program in older adults with knee osteoarthritis. The Fitness Arthritis and Seniors Trial (FAST). JAMA 277, 25–31.
Eyre, D. R. (2004). Collagens and cartilage matrix homeostasis. Clin. Orthop. Relat. Res. 427(Suppl.), S118–S122.
Fallah Mohammadi, M., Hajizadeh Moghaddam, A., and Mirkarimpur, H. (2013). The effects of a moderate exercise program on knee osteoarthritis in male wistar rats. Iran. J. Basic Med. Sci. 16, 683–688.
Fang, L., Lin, L., Lv, Y., Huang, Z., Lin, X., Wang, X., et al. (2021). The mechanism of aerobic exercise combined with glucosamine therapy and circUNK in improving knee osteoarthritis in rabbits. Life Sci. 275:119375. doi: 10.1016/j.lfs.2021.119375
Felson, D. T., Zhang, Y., Hannan, M. T., Naimark, A., Weissman, B., Aliabadi, P., et al. (1997). Risk factors for incident radiographic knee osteoarthritis in the elderly: the Framingham Study. Arthritis Rheum. 40, 728–733.
Fernandes, L., Roos, E. M., Overgaard, S., Villadsen, A., and Søgaard, R. (2017). Supervised neuromuscular exercise prior to hip and knee replacement: 12-month clinical effect and cost-utility analysis alongside a randomised controlled trial. BMC Musculoskelet. Disord. 18:5. doi: 10.1186/s12891-016-1369-0
Ferraz, R. B., Gualano, B., Rodrigues, R., Kurimori, C. O., Fuller, R., Lima, F. R., et al. (2018). Benefits of resistance training with blood flow restriction in knee osteoarthritis. Med. Sci. Sports Exerc. 50, 897–905. doi: 10.1249/MSS.0000000000001530
Foley, A., Halbert, J., Hewitt, T., and Crotty, M. (2003). Does hydrotherapy improve strength and physical function in patients with osteoarthritis–a randomised controlled trial comparing a gym based and a hydrotherapy based strengthening programme. Ann. Rheum. Dis. 62, 1162–1167.
Franciozi, C. E. S., Tarini, V. A. F., Reginato, R. D., Gonçalves, P. R. S., Medeiros, V. P., Ferretti, M., et al. (2013). Gradual strenuous running regimen predisposes to osteoarthritis due to cartilage cell death and altered levels of glycosaminoglycans. Osteoarthritis Cartilage 21, 965–972. doi: 10.1016/j.joca.2013.04.007
Galois, L., Etienne, S., Grossin, L., Watrin-Pinzano, A., Cournil-Henrionnet, C., Loeuille, D., et al. (2004). Dose-response relationship for exercise on severity of experimental osteoarthritis in rats: a pilot study. Osteoarthritis Cartilage 12, 779–786.
Gianni, L., Pienkowski, T., Im, Y.-H., Tseng, L.-M., Liu, M.-C., Lluch, A., et al. (2016). 5-year analysis of neoadjuvant pertuzumab and trastuzumab in patients with locally advanced, inflammatory, or early-stage HER2-positive breast cancer (NeoSphere): a multicentre, open-label, phase 2 randomised trial. Lancet Oncol. 17, 791–800. doi: 10.1016/S1470-2045(16)00163-7
CrossRef Full Text | Google Scholar
Goh, S.-L., Persson, M. S. M., Stocks, J., Hou, Y., Welton, N. J., Lin, J., et al. (2019). Relative efficacy of different exercises for pain, function, performance and quality of life in knee and hip osteoarthritis: systematic review and network meta-analysis. Sports Med. 49, 743–761. doi: 10.1007/s40279-019-01082-0
Goldring, M. B., and Goldring, S. R. (2007). Osteoarthritis. J. Cell. Physiol. 213, 626–634.
Goldring, M. B., and Otero, M. (2011). Inflammation in osteoarthritis. Curr. Opin. Rheumatol. 23, 471–478. doi: 10.1097/BOR.0b013e328349c2b1
Gracey, E., Burssens, A., Cambré, I., Schett, G., Lories, R., McInnes, I. B., et al. (2020). Tendon and ligament mechanical loading in the pathogenesis of inflammatory arthritis. Nat. Rev. Rheumatol. 16, 193–207. doi: 10.1038/s41584-019-0364-x
Griffin, T. M., Batushansky, A., Hudson, J., and Lopes, E. B. P. (2020). Correlation network analysis shows divergent effects of a long-term, high-fat diet and exercise on early stage osteoarthritis phenotypes in mice. J. Sport Health Sci. 9, 119–131. doi: 10.1016/j.jshs.2019.05.008
Griffin, T. M., Huebner, J. L., Kraus, V. B., Yan, Z., and Guilak, F. (2012). Induction of osteoarthritis and metabolic inflammation by a very high-fat diet in mice: effects of short-term exercise. Arthritis Rheum. 64, 443–453. doi: 10.1002/art.33332
Guan, Y.-J., Yang, X., Wei, L., and Chen, Q. (2011). MiR-365: a mechanosensitive microRNA stimulates chondrocyte differentiation through targeting histone deacetylase 4. FASEB J. 25, 4457–4466. doi: 10.1096/fj.11-185132
Guo, J., Chung, U.-I., Kondo, H., Bringhurst, F. R., and Kronenberg, H. M. (2002). The PTH/PTHrP receptor can delay chondrocyte hypertrophy in vivo without activating phospholipase C. Dev. Cell 3, 183–194.
Guo, Y.-F., Su, T., Yang, M., Li, C.-J., Guo, Q., Xiao, Y., et al. (2021). The role of autophagy in bone homeostasis. J. Cell. Physiol. 236, 4152–4173. doi: 10.1002/jcp.30111
Hao, X., Wang, S., Zhang, J., and Xu, T. (2021). Effects of body weight-supported treadmill training on cartilage-subchondral bone unit in the rat model of posttraumatic osteoarthritis. J. Orthop. Res. 39, 1227–1235. doi: 10.1002/jor.24791
Hashimoto, S., Ochs, R. L., Komiya, S., and Lotz, M. (1998). Linkage of chondrocyte apoptosis and cartilage degradation in human osteoarthritis. Arthritis Rheum. 41, 1632–1638.
He, Y., Wang, W., Xu, X., Yang, B., Yu, X., Wu, Y., et al. (2021). Mettl3 inhibits the apoptosis and autophagy of chondrocytes in inflammation through mediating Bcl2 stability via Ythdf1-mediated mA modification. Bone 154:116182. doi: 10.1016/j.bone.2021.116182
He, Z., Li, H., Han, X., Zhou, F., Du, J., Yang, Y., et al. (2020). Irisin inhibits osteocyte apoptosis by activating the Erk signaling pathway in vitro and attenuates ALCT-induced osteoarthritis in mice. Bone 141:115573. doi: 10.1016/j.bone.2020.115573
Héraud, F., Héraud, A., and Harmand, M. F. (2000). Apoptosis in normal and osteoarthritic human articular cartilage. Ann. Rheum. Dis. 59, 959–965.
Hinman, R. S., Heywood, S. E., and Day, A. R. (2007). Aquatic physical therapy for hip and knee osteoarthritis: results of a single-blind randomized controlled trial. Phys. Ther. 87, 32–43.
Hiyama, Y., Yamada, M., Kitagawa, A., Tei, N., and Okada, S. (2012). A four-week walking exercise programme in patients with knee osteoarthritis improves the ability of dual-task performance: a randomized controlled trial. Clin. Rehabil. 26, 403–412. doi: 10.1177/0269215511421028
Holm, P. M., Petersen, K. K., Wernbom, M., Schrøder, H. M., Arendt-Nielsen, L., and Skou, S. T. (2021). Strength training in addition to neuromuscular exercise and education in individuals with knee osteoarthritis-the effects on pain and sensitization. Eur. J. Pain 25, 1898–1911. doi: 10.1002/ejp.1796
Holyoak, D. T., Chlebek, C., Kim, M. J., Wright, T. M., Otero, M., and van der Meulen, M. C. H. (2019). Low-level cyclic tibial compression attenuates early osteoarthritis progression after joint injury in mice. Osteoarthritis Cartilage 27, 1526–1536. doi: 10.1016/j.joca.2019.06.005
Hong, E., and Reddi, A. H. (2012). MicroRNAs in chondrogenesis, articular cartilage, and osteoarthritis: implications for tissue engineering. Tissue Eng. Part B Rev. 18, 445–453. doi: 10.1089/ten.TEB.2012.0116
Hong, Y., Kim, H., Lee, Y., Lee, S., Kim, K., Jin, Y., et al. (2014). Salutary effects of melatonin combined with treadmill exercise on cartilage damage. J. Pineal Res. 57, 53–66. doi: 10.1111/jpi.12143
Houard, X., Goldring, M. B., and Berenbaum, F. (2013). Homeostatic mechanisms in articular cartilage and role of inflammation in osteoarthritis. Curr. Rheumatol. Rep. 15:375. doi: 10.1007/s11926-013-0375-6
Hsieh, Y.-L., and Yang, C.-C. (2018). Early intervention of swimming exercises attenuate articular cartilage destruction in a rat model of anterior cruciate ligament and meniscus knee injuries. Life Sci. 212, 267–274. doi: 10.1016/j.lfs.2018.10.013
Hsu, Y.-I., Chen, Y.-C., Lee, C.-L., and Chang, N.-J. (2021). Effects of diet control and telemedicine-based resistance exercise intervention on patients with obesity and knee osteoarthritis: a randomized control trial. Int. J. Environ. Res. Public Health 18:7744. doi: 10.3390/ijerph18157744
Huang, J., Zhao, L., Fan, Y., Liao, L., Ma, P. X., Xiao, G., et al. (2019). The microRNAs miR-204 and miR-211 maintain joint homeostasis and protect against osteoarthritis progression. Nat. Commun. 10:2876. doi: 10.1038/s41467-019-10753-5
Huang, L., Guo, B., Xu, F., and Zhao, J. (2018). Effects of quadriceps functional exercise with isometric contraction in the treatment of knee osteoarthritis. Int. J. Rheum. Dis. 21, 952–959. doi: 10.1111/1756-185X.13082
Hwang, H. S., and Kim, H. A. (2015). Chondrocyte apoptosis in the pathogenesis of osteoarthritis. Int. J. Mol. Sci. 16, 26035–26054. doi: 10.3390/ijms161125943
Iijima, H., Aoyama, T., Ito, A., Tajino, J., Yamaguchi, S., Nagai, M., et al. (2016). Exercise intervention increases expression of bone morphogenetic proteins and prevents the progression of cartilage-subchondral bone lesions in a post-traumatic rat knee model. Osteoarthritis Cartilage 24, 1092–1102. doi: 10.1016/j.joca.2016.01.006
Iijima, H., Aoyama, T., Ito, A., Yamaguchi, S., Nagai, M., Tajino, J., et al. (2015). Effects of short-term gentle treadmill walking on subchondral bone in a rat model of instability-induced osteoarthritis. Osteoarthritis Cartilage 23, 1563–1574. doi: 10.1016/j.joca.2015.04.015
Iijima, H., Ito, A., Nagai, M., Tajino, J., Yamaguchi, S., Kiyan, W., et al. (2017). Physiological exercise loading suppresses post-traumatic osteoarthritis progression via an increase in bone morphogenetic proteins expression in an experimental rat knee model. Osteoarthritis Cartilage 25, 964–975. doi: 10.1016/j.joca.2016.12.008
Jansen, M. J., Viechtbauer, W., Lenssen, A. F., Hendriks, E. J. M., and de Bie, R. A. (2011). Strength training alone, exercise therapy alone, and exercise therapy with passive manual mobilisation each reduce pain and disability in people with knee osteoarthritis: a systematic review. J. Physiother. 57, 11–20. doi: 10.1016/S1836-9553(11)70002-9
Jia, Z., and Wei, Q.-J. (2021). CircRNA-MSR Regulates LPS-Induced C28/I2 Chondrocyte Injury through miR-643/MAP2K6 Signaling Pathway. Cartilage 13(2_Suppl), 785S–795S. doi: 10.1177/19476035211044826
Jorge, R. T. B., Souza, M. C. D., Chiari, A., Jones, A., Fernandes, A. D. R. C., Lombardi Júnior, I., et al. (2015). Progressive resistance exercise in women with osteoarthritis of the knee: a randomized controlled trial. Clin. Rehabil. 29, 234–243. doi: 10.1177/0269215514540920
Jusic, A., and Devaux, Y. (2020). Mitochondrial noncoding RNA-regulatory network in cardiovascular disease. Basic Res. Cardiol. 115:23. doi: 10.1007/s00395-020-0783-5
Kabiri, S., Halabchi, F., Angoorani, H., and Yekaninejad, S. (2018). Comparison of three modes of aerobic exercise combined with resistance training on the pain and function of patients with knee osteoarthritis: a randomized controlled trial. Phys. Ther. Sport 32, 22–28. doi: 10.1016/j.ptsp.2018.04.001
Kapoor, M., Martel-Pelletier, J., Lajeunesse, D., Pelletier, J.-P., and Fahmi, H. (2011). Role of proinflammatory cytokines in the pathophysiology of osteoarthritis. Nat. Rev. Rheumatol. 7, 33–42. doi: 10.1038/nrrheum.2010.196
Karreth, F. A., and Pandolfi, P. P. (2013). ceRNA cross-talk in cancer: When ce-bling rivalries go awry. Cancer Discov. 3, 1113–1121. doi: 10.1158/2159-8290.CD-13-0202
Kim, D. Y., Taylor, H. W., Moore, R. M., Paulsen, D. B., and Cho, D. Y. (2003). Articular chondrocyte apoptosis in equine osteoarthritis. Vet. J. 166, 52–57.
Kongara, S., and Karantza, V. (2012). The interplay between autophagy and ROS in tumorigenesis. Front. Oncol. 2:171. doi: 10.3389/fonc.2012.00171
Krauß, I., Steinhilber, B., Haupt, G., Miller, R., Martus, P., and Janßen, P. (2014). Exercise therapy in hip osteoarthritis–a randomized controlled trial. Dtsch. Arztebl. Int. 111, 592–599. doi: 10.3238/arztebl.2014.0592
Kuntz, A. B., Chopp-Hurley, J. N., Brenneman, E. C., Karampatos, S., Wiebenga, E. G., Adachi, J. D., et al. (2018). Efficacy of a biomechanically-based yoga exercise program in knee osteoarthritis: a randomized controlled trial. PLoS One 13:e0195653. doi: 10.1371/journal.pone.0195653
Kuptniratsaikul, V., Kittichaikarn, C., Suntornpiyapan, P., Kovintaset, K., and Inthibal, S. (2019). Is four-week underwater treadmill exercise regimen compared to home exercise efficacious for pain relief and functional improvement in obese patients with knee osteoarthritis? A randomized controlled trial. Clin. Rehabil. 33, 85–93. doi: 10.1177/0269215518792041
Lai, Z., Zhang, Y., Lee, S., and Wang, L. (2018). Effects of strength exercise on the knee and ankle proprioception of individuals with knee osteoarthritis. Res. Sports Med. 26, 138–146. doi: 10.1080/15438627.2018.1431541
León-Ballesteros, S., Espinosa-Morales, R., Clark-Peralta, P., Gómez-Pineda, A. G., and Guadarrama-Becerril, J. H. (2020). Kinesiotape and quadriceps strengthening with elastic band in women with knee osteoarthritis and overweight or obesity. A randomized clinical trial. Reumatol. Clin. 16, 11–16. doi: 10.1016/j.reuma.2018.03.001
Li, Z., Huang, Z., Zhang, H., Lu, J., Wei, Y., Yang, Y., et al. (2021). IRE1-mTOR-PERK Axis coordinates autophagy and er stress-apoptosis induced by p2x7-mediated ca influx in osteoarthritis. Front. Cell Dev. Biol. 9:695041. doi: 10.3389/fcell.2021.695041
Li, K., Liu, A., Zong, W., Dai, L., Liu, Y., Luo, R., et al. (2021). Moderate exercise ameliorates osteoarthritis by reducing lipopolysaccharides from gut microbiota in mice. Saudi J. Biol. Sci. 28, 40–49. doi: 10.1016/j.sjbs.2020.08.027
Li, R., Chen, H., Feng, J., Xiao, Y., Zhang, H., Lam, C. W.-K., et al. (2020). Effectiveness of traditional chinese exercise for symptoms of knee osteoarthritis: a systematic review and meta-analysis of randomized controlled trials. Int. J. Environ. Res. Public Health 17:7873. doi: 10.3390/ijerph17217873
Li, Y.-S., Zhang, F.-J., Zeng, C., Luo, W., Xiao, W.-F., Gao, S.-G., et al. (2016). Autophagy in osteoarthritis. Joint Bone Spine 83, 143–148. doi: 10.1016/j.jbspin.2015.06.009
Li, Z.-C., Xiao, J., Peng, J.-L., Chen, J.-W., Ma, T., Cheng, G.-Q., et al. (2014). Functional annotation of rheumatoid arthritis and osteoarthritis associated genes by integrative genome-wide gene expression profiling analysis. PLoS One 9:e85784. doi: 10.1371/journal.pone.0085784
Lieberthal, J., Sambamurthy, N., and Scanzello, C. R. (2015). Inflammation in joint injury and post-traumatic osteoarthritis. Osteoarthritis Cartilage 23, 1825–1834. doi: 10.1016/j.joca.2015.08.015
Liu, Q., Hu, X., Zhang, X., Dai, L., Duan, X., Zhou, C., et al. (2016). The TMSB4 Pseudogene LncRNA functions as a competing endogenous RNA to promote cartilage degradation in human osteoarthritis. Mol. Ther. 24, 1726–1733. doi: 10.1038/mt.2016.151
Liu, S.-S., Zhou, P., and Zhang, Y. (2016). Abnormal expression of key genes and proteins in the canonical Wnt/β-catenin pathway of articular cartilage in a rat model of exercise-induced osteoarthritis. Mol. Med. Rep. 13, 1999–2006. doi: 10.3892/mmr.2016.4798
Liu, Q., Zhang, X., Hu, X., Yuan, L., Cheng, J., Jiang, Y., et al. (2017). Emerging Roles of circRNA related to the mechanical stress in human cartilage degradation of osteoarthritis. Mol. Ther. Nucleic Acids 7, 223–230. doi: 10.1016/j.omtn.2017.04.004
Liu-Bryan, R. (2013). Synovium and the innate inflammatory network in osteoarthritis progression. Curr. Rheumatol. Rep. 15:323. doi: 10.1007/s11926-013-0323-5
Loeser, R. F., Goldring, S. R., Scanzello, C. R., and Goldring, M. B. (2012). Osteoarthritis: a disease of the joint as an organ. Arthritis Rheum. 64, 1697–1707. doi: 10.1002/art.34453
Lorenzo, P., Bayliss, M. T., and Heinegård, D. (2004). Altered patterns and synthesis of extracellular matrix macromolecules in early osteoarthritis. Matrix Biol. 23, 381–391.
Lu, J., Feng, X., Zhang, H., Wei, Y., Yang, Y., Tian, Y., et al. (2021). Maresin-1 suppresses IL-1β-induced MMP-13 secretion by activating the PI3K/AKT pathway and inhibiting the NF-κB pathway in synovioblasts of an osteoarthritis rat model with treadmill exercise. Connect. Tissue Res. 62, 508–518. doi: 10.1080/03008207.2020.1780218
Lun, V., Marsh, A., Bray, R., Lindsay, D., and Wiley, P. (2015). Efficacy of hip strengthening exercises compared with leg strengthening exercises on knee pain, function, and quality of life in patients with knee osteoarthritis. Clin. J. Sport Med. 25, 509–517. doi: 10.1097/JSM.0000000000000170
Lund, H., Weile, U., Christensen, R., Rostock, B., Downey, A., Bartels, E. M., et al. (2008). A randomized controlled trial of aquatic and land-based exercise in patients with knee osteoarthritis. J. Rehabil. Med. 40, 137–144. doi: 10.2340/16501977-0134
Luo, Y., Sinkeviciute, D., He, Y., Karsdal, M., Henrotin, Y., Mobasheri, A., et al. (2017). The minor collagens in articular cartilage. Protein Cell 8, 560–572. doi: 10.1007/s13238-017-0377-7
Ma, Z., and Ji, J. (2020). N6-methyladenosine (m6A) RNA modification in cancer stem cells. Stem Cells. doi: 10.1002/stem.3279 [Epub ahead of print].
Malemud, C. J. (2017). Matrix metalloproteinases and synovial joint pathology. Prog. Mol. Biol. Transl. Sci. 148, 305–325. doi: 10.1016/bs.pmbts.2017.03.003
Malfait, A. M. (2016). Osteoarthritis year in review 2015: biology. Osteoarthritis Cartilage 24, 21–26. doi: 10.1016/j.joca.2015.09.010
Martins, J. B., Mendonça, V. A., Aguiar, G. C., da Fonseca, S. F., Dos Santos, J. M., Tossige-Gomes, R., et al. (2019). Effect of a moderate-intensity aerobic training on joint biomarkers and functional adaptations in rats subjected to induced knee osteoarthritis. Front. Physiol. 10:1168. doi: 10.3389/fphys.2019.01168
Mathew, R., Karp, C. M., Beaudoin, B., Vuong, N., Chen, G., Chen, H.-Y., et al. (2009). Autophagy suppresses tumorigenesis through elimination of p62. Cell 137, 1062–1075. doi: 10.1016/j.cell.2009.03.048
Maurer, B. T., Stern, A. G., Kinossian, B., Cook, K. D., and Schumacher, H. R. (1999). Osteoarthritis of the knee: isokinetic quadriceps exercise versus an educational intervention. Arch. Phys. Med. Rehabil. 80, 1293–1299.
Messier, S. P., Mihalko, S. L., Beavers, D. P., Nicklas, B. J., DeVita, P., Carr, J. J., et al. (2021). Effect of high-intensity strength training on knee pain and knee joint compressive forces among adults with knee osteoarthritis: the START randomized clinical trial. JAMA 325, 646–657. doi: 10.1001/jama.2021.0411
Milares, L. P., Assis, L., Siqueira, A., Claudino, V., Domingos, H., Almeida, T., et al. (2016). Effectiveness of an aquatic exercise program and low-level laser therapy on articular cartilage in an experimental model of osteoarthritis in rats. Connect. Tissue Res. 57, 398–407. doi: 10.1080/03008207.2016.1193174
Mobasheri, A., Rayman, M. P., Gualillo, O., Sellam, J., van der Kraan, P., and Fearon, U. (2017). The role of metabolism in the pathogenesis of osteoarthritis. Nat. Rev. Rheumatol. 13, 302–311. doi: 10.1038/nrrheum.2017.50
Multanen, J., Rantalainen, T., Kautiainen, H., Ahola, R., Jämsä, T., Nieminen, M. T., et al. (2017). Effect of progressive high-impact exercise on femoral neck structural strength in postmenopausal women with mild knee osteoarthritis: a 12-month RCT. Osteoporos. Int. 28, 1323–1333. doi: 10.1007/s00198-016-3875-1
Munukka, M., Waller, B., Häkkinen, A., Nieminen, M. T., Lammentausta, E., Kujala, U. M., et al. (2020). Effects of progressive aquatic resistance training on symptoms and quality of life in women with knee osteoarthritis: a secondary analysis. Scand. J. Med. Sci. Sports 30, 1064–1072. doi: 10.1111/sms.13630
Munukka, M., Waller, B., Rantalainen, T., Häkkinen, A., Nieminen, M. T., Lammentausta, E., et al. (2016). Efficacy of progressive aquatic resistance training for tibiofemoral cartilage in postmenopausal women with mild knee osteoarthritis: a randomised controlled trial. Osteoarthritis Cartilage 24, 1708–1717. doi: 10.1016/j.joca.2016.05.007
Musumeci, G., Castrogiovanni, P., Trovato, F. M., Imbesi, R., Giunta, S., Szychlinska, M. A., et al. (2015). Physical activity ameliorates cartilage degeneration in a rat model of aging: a study on lubricin expression. Scand. J. Med. Sci. Sports 25, e222–e230. doi: 10.1111/sms.12290
Musumeci, G., Loreto, C., Carnazza, M. L., and Martinez, G. (2011). Characterization of apoptosis in articular cartilage derived from the knee joints of patients with osteoarthritis. Knee Surg. Sports Traumatol. Arthrosc. 19, 307–313.
Musumeci, G., Loreto, C., Leonardi, R., Castorina, S., Giunta, S., Carnazza, M. L., et al. (2013). The effects of physical activity on apoptosis and lubricin expression in articular cartilage in rats with glucocorticoid-induced osteoporosis. J. Bone Miner. Metab. 31, 274–284. doi: 10.1007/s00774-012-0414-9
Na, S. S., Kim, S. G., Yong, M. S., and Hwangbo, G. (2014). Study of treadmill exercise effect on rats with osteoarthritis using proteomic analysis. J. Phys. Ther. Sci. 26, 487–490. doi: 10.1589/jpts.26.487
Nery, M., Natour, J., Jennings, F., Fernandes, A. D. R. C., Souza, M. C., and Jones, A. (2021). Effects of a progressive resistance exercise program in patients with hand osteoarthritis: a randomized, controlled trial with a blinded assessor. Clin. Rehabil. 35, 1757–1767. doi: 10.1177/02692155211030622
Norrdin, R. W., Kawcak, C. E., Capwell, B. A., and McIlwraith, C. W. (1998). Subchondral bone failure in an equine model of overload arthrosis. Bone 22, 133–139.
Ojoawo, A. O., Olaogun, M. O. B., and Hassan, M. A. (2016). Comparative effects of proprioceptive and isometric exercises on pain intensity and difficulty in patients with knee osteoarthritis: a randomised control study. Technol. Health Care 24, 853–863.
Oka, Y., Murata, K., Kano, T., Ozone, K., Arakawa, K., Kokubun, T., et al. (2019). Impact of controlling abnormal joint movement on the effectiveness of subsequent exercise intervention in mouse models of early knee osteoarthritis. Cartilage 13, (2_Suppl), 1334S–1344S. doi: 10.1177/1947603519885007
Oka, Y., Murata, K., Ozone, K., Kano, T., Minegishi, Y., Kuro-Nakajima, A., et al. (2021). Treadmill exercise after controlled abnormal joint movement inhibits cartilage degeneration and synovitis. Life 11:303. doi: 10.3390/life11040303
Oliveira, A. M. I. D., Peccin, M. S., Silva, K. N. G. D., Teixeira, L. E. P. D. P., and Trevisani, V. F. M. (2012). Impact of exercise on the functional capacity and pain of patients with knee osteoarthritis: a randomized clinical trial. Rev. Bras. Reumatol. 52, 876–882.
Pap, T., Dankbar, B., Wehmeyer, C., Korb-Pap, A., and Sherwood, J. (2020). Synovial fibroblasts and articular tissue remodelling: role and mechanisms. Semin. Cell Dev. Biol. 101, 140–145. doi: 10.1016/j.semcdb.2019.12.006
Pedersen, B. K., and Saltin, B. (2015). Exercise as medicine - evidence for prescribing exercise as therapy in 26 different chronic diseases. Scand. J. Med. Sci. Sports 25(Suppl.), 3. doi: 10.1111/sms.12581
Peng, M.-S., Wang, R., Wang, Y.-Z., Chen, C.-C., Wang, J., Liu, X.-C., et al. (2022). Efficacy of therapeutic aquatic exercise vs physical therapy modalities for patients with chronic low back pain: a randomized clinical trial. JAMA Netw. Open 5:e2142069. doi: 10.1001/jamanetworkopen.2021.42069
Penninx, B. W. J. H., Rejeski, W. J., Pandya, J., Miller, M. E., Di Bari, M., Applegate, W. B., et al. (2002). Exercise and depressive symptoms: a comparison of aerobic and resistance exercise effects on emotional and physical function in older persons with high and low depressive symptomatology. J. Gerontol. B Psychol. Sci. Soc. Sci. 57, 124–132.
Pérez-García, S., Carrión, M., Gutiérrez-Cañas, I., Villanueva-Romero, R., Castro, D., Martínez, C., et al. (2019). Profile of matrix-remodeling proteinases in osteoarthritis: impact of fibronectin. Cells 9:40. doi: 10.3390/cells9010040
Pisters, M. F., Veenhof, C., de Bakker, D. H., Schellevis, F. G., and Dekker, J. (2010). Behavioural graded activity results in better exercise adherence and more physical activity than usual care in people with osteoarthritis: a cluster-randomised trial. J. Physiother. 56, 41–47.
Qian, J., Liang, J., Wang, Y., and Wang, H. (2014). Effect of passive motion on articular cartilage in rat osteoarthritis. Exp. Ther. Med. 8, 377–383.
Rabe, K. G., Matsuse, H., Jackson, A., and Segal, N. A. (2018). Evaluation of the combined application of neuromuscular electrical stimulation and volitional contractions on thigh muscle strength, knee pain, and physical performance in women at risk for knee osteoarthritis: a randomized controlled trial. PM R 10, 1301–1310. doi: 10.1016/j.pmrj.2018.05.014
Rahmati, M., Nalesso, G., Mobasheri, A., and Mozafari, M. (2017). Aging and osteoarthritis: central role of the extracellular matrix. Ageing Res. Rev. 40, 20–30. doi: 10.1016/j.arr.2017.07.004
Rewald, S., Lenssen, A. F. T., Emans, P. J., de Bie, R. A., van Breukelen, G., and Mesters, I. (2020). Aquatic cycling improves knee pain and physical functioning in patients with knee osteoarthritis: a randomized controlled trial. Arch. Phys. Med. Rehabil. 101, 1288–1295. doi: 10.1016/j.apmr.2019.12.023
Reynard, L. N. (2017). Analysis of genetics and DNA methylation in osteoarthritis: What have we learnt about the disease? Semin. Cell Dev. Biol. 62, 57–66. doi: 10.1016/j.semcdb.2016.04.017
Rim, Y. A., Nam, Y., and Ju, J. H. (2020). The role of chondrocyte hypertrophy and senescence in osteoarthritis initiation and progression. Int. J. Mol. Sci. 21:2358. doi: 10.3390/ijms21072358
Rios, J. L., Bomhof, M. R., Reimer, R. A., Hart, D. A., Collins, K. H., and Herzog, W. (2019). Protective effect of prebiotic and exercise intervention on knee health in a rat model of diet-induced obesity. Sci. Rep. 9:3893. doi: 10.1038/s41598-019-40601-x
Rios, J. L., Hart, D. A., Reimer, R. A., and Herzog, W. (2020). Prebiotic and exercise do not alter knee osteoarthritis in a rat model of established obesity. Cartilage 13(2_Suppl), 1456S–1466S. doi: 10.1177/1947603520959399
Rojas-Ortega, M., Cruz, R., Vega-López, M. A., Cabrera-González, M., Hernández-Hernández, J. M., Lavalle-Montalvo, C., et al. (2015). Exercise modulates the expression of IL-1β and IL-10 in the articular cartilage of normal and osteoarthritis-induced rats. Pathol. Res. Pract. 211, 435–443. doi: 10.1016/j.prp.2015.01.008
Rosshirt, N., Hagmann, S., Tripel, E., Gotterbarm, T., Kirsch, J., Zeifang, F., et al. (2019). A predominant Th1 polarization is present in synovial fluid of end-stage osteoarthritic knee joints: analysis of peripheral blood, synovial fluid and synovial membrane. Clin. Exp. Immunol. 195, 395–406. doi: 10.1111/cei.13230
Ruegsegger, G. N., and Booth, F. W. (2018). Health benefits of exercise. Cold Spring Harb. Perspect. Med. 8:a029694. doi: 10.1101/cshperspect.a029694
Sadeghi, H., and Jehu, D. A. (2022). Exergaming to improve physical, psychological and cognitive health among home office workers: a COVID-19 pandemic commentary. Work 71, 13–17. doi: 10.3233/WOR-211000
Samut, G., Dinçer, F., and Özdemir, O. (2015). The effect of isokinetic and aerobic exercises on serum interleukin-6 and tumor necrosis factor alpha levels, pain, and functional activity in patients with knee osteoarthritis. Mod. Rheumatol. 25, 919–924. doi: 10.3109/14397595.2015.1038425
Sang, W., Xue, S., Jiang, Y., Lu, H., Zhu, L., Wang, C., et al. (2021). METTL3 involves the progression of osteoarthritis probably by affecting ECM degradation and regulating the inflammatory response. Life Sci. 278:119528. doi: 10.1016/j.lfs.2021.119528
Sasaki, H., Takayama, K., Matsushita, T., Ishida, K., Kubo, S., Matsumoto, T., et al. (2012). Autophagy modulates osteoarthritis-related gene expression in human chondrocytes. Arthritis Rheum. 64, 1920–1928. doi: 10.1002/art.34323
Scanzello, C. R. (2017a). Chemokines and inflammation in osteoarthritis: insights from patients and animal models. J. Orthop. Res. 35, 735–739. doi: 10.1002/jor.23471
Scanzello, C. R. (2017b). Role of low-grade inflammation in osteoarthritis. Curr. Opin. Rheumatol. 29, 79–85.
Scanzello, C. R., and Goldring, S. R. (2012). The role of synovitis in osteoarthritis pathogenesis. Bone 51, 249–257. doi: 10.1016/j.bone.2012.02.012
Scanzello, C. R., McKeon, B., Swaim, B. H., DiCarlo, E., Asomugha, E. U., Kanda, V., et al. (2011). Synovial inflammation in patients undergoing arthroscopic meniscectomy: molecular characterization and relationship to symptoms. Arthritis Rheum. 63, 391–400. doi: 10.1002/art.30137
Setayeshmehr, M., Esfandiari, E., Rafieinia, M., Hashemibeni, B., Taheri-Kafrani, A., Samadikuchaksaraei, A., et al. (2019). Hybrid and composite scaffolds based on extracellular matrices for cartilage tissue engineering. Tissue Eng. Part B Rev. 25, 202–224. doi: 10.1089/ten.TEB.2018.0245
Sevick, M. A., Bradham, D. D., Muender, M., Chen, G. J., Enarson, C., Dailey, M., et al. (2000). Cost-effectiveness of aerobic and resistance exercise in seniors with knee osteoarthritis. Med. Sci. Sports Exerc. 32, 1534–1540.
Silva, L. E., Valim, V., Pessanha, A. P. C., Oliveira, L. M., Myamoto, S., Jones, A., et al. (2008). Hydrotherapy versus conventional land-based exercise for the management of patients with osteoarthritis of the knee: a randomized clinical trial. Phys. Ther. 88, 12–21.
Simão, A. P., Avelar, N. C., Tossige-Gomes, R., Neves, C. D., Mendonça, V. A., Miranda, A. S., et al. (2012). Functional performance and inflammatory cytokines after squat exercises and whole-body vibration in elderly individuals with knee osteoarthritis. Arch. Phys. Med. Rehabil. 93, 1692–1700. doi: 10.1016/j.apmr.2012.04.017
Skou, S. T., Pedersen, B. K., Abbott, J. H., Patterson, B., and Barton, C. (2018). Physical activity and exercise therapy benefit more than just symptoms and impairments in people with hip and knee osteoarthritis. J. Orthop. Sports Phys. Ther. 48, 439–447. doi: 10.2519/jospt.2018.7877
Song, R., Lee, E.-O., Lam, P., and Bae, S.-C. (2003). Effects of tai chi exercise on pain, balance, muscle strength, and perceived difficulties in physical functioning in older women with osteoarthritis: a randomized clinical trial. J. Rheumatol. 30, 2039–2044.
Sowers, M., Karvonen-Gutierrez, C. A., Jacobson, J. A., Jiang, Y., and Yosef, M. (2011). Associations of anatomical measures from MRI with radiographically defined knee osteoarthritis score, pain, and physical functioning. J. Bone Joint Surg. Am. 93, 241–251. doi: 10.2106/JBJS.I.00667
Spahn, G., Hofmann, G. O., von Engelhardt, L. V., Li, M., Neubauer, H., and Klinger, H. M. (2013). The impact of a high tibial valgus osteotomy and unicondylar medial arthroplasty on the treatment for knee osteoarthritis: a meta-analysis. Knee Surg. Sports Traumatol. Arthrosc. 21, 96–112. doi: 10.1007/s00167-011-1751-2
Steinhilber, B., Haupt, G., Miller, R., Janssen, P., and Krauss, I. (2017). Exercise therapy in patients with hip osteoarthritis: effect on hip muscle strength and safety aspects of exercise-results of a randomized controlled trial. Mod. Rheumatol. 27, 493–502. doi: 10.1080/14397595.2016.1213940
Sun, X., Xiao, L., Chen, J., Chen, X., Chen, X., Yao, S., et al. (2020). DNA methylation is involved in the pathogenesis of osteoarthritis by regulating expression and CtBP-mediated signaling. Int. J. Biol. Sci. 16, 994–1009. doi: 10.7150/ijbs.39945
Suri, S., and Walsh, D. A. (2012). Osteochondral alterations in osteoarthritis. Bone 51, 204–211. doi: 10.1016/j.bone.2011.10.010
Suzuki, Y., Iijima, H., Tashiro, Y., Kajiwara, Y., Zeidan, H., Shimoura, K., et al. (2019). Home exercise therapy to improve muscle strength and joint flexibility effectively treats pre-radiographic knee OA in community-dwelling elderly: a randomized controlled trial. Clin. Rheumatol. 38, 133–141. doi: 10.1007/s10067-018-4263-3
Swingler, T. E., Wheeler, G., Carmont, V., Elliott, H. R., Barter, M. J., Abu-Elmagd, M., et al. (2012). The expression and function of microRNAs in chondrogenesis and osteoarthritis. Arthritis Rheum. 64, 1909–1919. doi: 10.1002/art.34314
Szychlinska, M. A., Castrogiovanni, P., Trovato, F. M., Nsir, H., Zarrouk, M., Lo Furno, D., et al. (2019). Physical activity and Mediterranean diet based on olive tree phenolic compounds from two different geographical areas have protective effects on early osteoarthritis, muscle atrophy and hepatic steatosis. Eur. J. Nutr. 58, 565–581. doi: 10.1007/s00394-018-1632-2
Taglietti, M., Facci, L. M., Trelha, C. S., de Melo, F. C., da Silva, D. W., Sawczuk, G., et al. (2018). Effectiveness of aquatic exercises compared to patient-education on health status in individuals with knee osteoarthritis: a randomized controlled trial. Clin. Rehabil. 32, 766–776. doi: 10.1177/0269215517754240
Theocharis, A. D., Manou, D., and Karamanos, N. K. (2019). The extracellular matrix as a multitasking player in disease. FEBS J. 286, 2830–2869. doi: 10.1111/febs.14818
Thomas, C. M., Whittles, C. E., Fuller, C. J., and Sharif, M. (2011). Variations in chondrocyte apoptosis may explain the increased prevalence of osteoarthritis in some joints. Rheumatol. Int. 31, 1341–1348. doi: 10.1007/s00296-010-1471-9
Tian, Y., Gou, J., Zhang, H., Lu, J., Jin, Z., Jia, S., et al. (2021). The anti-inflammatory effects of 15-HETE on osteoarthritis during treadmill exercise. Life Sci. 273:119260. doi: 10.1016/j.lfs.2021.119260
Todd Allen, R., Robertson, C. M., Harwood, F. L., Sasho, T., Williams, S. K., Pomerleau, A. C., et al. (2004). Characterization of mature vs aged rabbit articular cartilage: analysis of cell density, apoptosis-related gene expression and mechanisms controlling chondrocyte apoptosis. Osteoarthritis Cartilage 12, 917–923.
Topp, R., Woolley, S., Hornyak, J., Khuder, S., and Kahaleh, B. (2002). The effect of dynamic versus isometric resistance training on pain and functioning among adults with osteoarthritis of the knee. Arch. Phys. Med. Rehabil. 83, 1187–1195.
Trans, T., Aaboe, J., Henriksen, M., Christensen, R., Bliddal, H., and Lund, H. (2009). Effect of whole body vibration exercise on muscle strength and proprioception in females with knee osteoarthritis. Knee 16, 256–261. doi: 10.1016/j.knee.2008.11.014
Tsauo, J.-Y., Cheng, P.-F., and Yang, R.-S. (2008). The effects of sensorimotor training on knee proprioception and function for patients with knee osteoarthritis: a preliminary report. Clin. Rehabil. 22, 448–457. doi: 10.1177/0269215507084597
Urnov, F. D. (2002). Methylation and the genome: the power of a small amendment. J. Nutr. 132(8 Suppl.), 2450S–2456S. doi: 10.1093/jn/132.8.2450S
Valdes, A. M., and Spector, T. D. (2010). The genetic epidemiology of osteoarthritis. Curr. Opin. Rheumatol. 22, 139–143. doi: 10.1097/BOR.0b013e3283367a6e
van den Bosch, M. H. J. (2019). Inflammation in osteoarthritis: Is it time to dampen the alarm(in) in this debilitating disease? Clin. Exp. Immunol. 195, 153–166. doi: 10.1111/cei.13237
van Saase, J. L., van Romunde, L. K., Cats, A., Vandenbroucke, J. P., and Valkenburg, H. A. (1989). Epidemiology of osteoarthritis: Zoetermeer survey. Comparison of radiological osteoarthritis in a Dutch population with that in 10 other populations. Ann. Rheum. Dis. 48, 271–280.
Vasilceac, F. A., Marqueti, R. D. C., Neto, I. V. D. S., Nascimento, D. D. C., Souza, M. C. D., Durigan, J. L. Q., et al. (2021). Resistance training decreases matrix metalloproteinase-2 activity in quadriceps tendon in a rat model of osteoarthritis. Braz. J. Phys. Ther. 25, 147–155. doi: 10.1016/j.bjpt.2020.03.002
Villadsen, A., Overgaard, S., Holsgaard-Larsen, A., Christensen, R., and Roos, E. M. (2014). Immediate efficacy of neuromuscular exercise in patients with severe osteoarthritis of the hip or knee: a secondary analysis from a randomized controlled trial. J. Rheumatol. 41, 1385–1394. doi: 10.3899/jrheum.130642
Vincent, K. R., Vasilopoulos, T., Montero, C., and Vincent, H. K. (2019). Eccentric and concentric resistance exercise comparison for knee osteoarthritis. Med. Sci. Sports Exerc. 51, 1977–1986. doi: 10.1249/MSS.0000000000002010
Vincent, K. R., and Vincent, H. K. (2020). Concentric and eccentric resistance training comparison on physical function and functional pain outcomes in knee osteoarthritis: a randomized controlled trial. Am. J. Phys. Med. Rehabil. 99, 932–940. doi: 10.1097/PHM.0000000000001450
Vincent, T. L. (2019). Mechanoflammation in osteoarthritis pathogenesis. Semin. Arthritis Rheum. 49, S36–S38. doi: 10.1016/j.semarthrit.2019.09.018
Waller, B., Munukka, M., Rantalainen, T., Lammentausta, E., Nieminen, M. T., Kiviranta, I., et al. (2017). Effects of high intensity resistance aquatic training on body composition and walking speed in women with mild knee osteoarthritis: a 4-month RCT with 12-month follow-up. Osteoarthritis Cartilage 25, 1238–1246. doi: 10.1016/j.joca.2017.02.800
Wang, F., Zhang, X., Tong, X., Zhang, M., Xing, F., Yang, K., et al. (2021). The effects on pain, physical function, and quality of life of quadriceps strengthening exercises combined with Baduanjin qigong in older adults with knee osteoarthritis: a quasi-experimental study. BMC Musculoskelet. Disord. 22:313. doi: 10.1186/s12891-021-04179-8
Wang, P., Yang, L., Li, H., Lei, Z., Yang, X., Liu, C., et al. (2016). Effects of whole-body vibration training with quadriceps strengthening exercise on functioning and gait parameters in patients with medial compartment knee osteoarthritis: a randomised controlled preliminary study. Physiotherapy 102, 86–92. doi: 10.1016/j.physio.2015.03.3720
Wang, T.-J., Belza, B., Elaine Thompson, F., Whitney, J. D., and Bennett, K. (2007). Effects of aquatic exercise on flexibility, strength and aerobic fitness in adults with osteoarthritis of the hip or knee. J. Adv. Nurs. 57, 141–152.
Wang, T.-J., Lee, S.-C., Liang, S.-Y., Tung, H.-H., Wu, S.-F. V., and Lin, Y.-P. (2011). Comparing the efficacy of aquatic exercises and land-based exercises for patients with knee osteoarthritis. J. Clin. Nurs. 20, 2609–2622. doi: 10.1111/j.1365-2702.2010.03675.x
Woodell-May, J. E., and Sommerfeld, S. D. (2020). Role of inflammation and the immune system in the progression of osteoarthritis. J. Orthop. Res. 38, 253–257. doi: 10.1002/jor.24457
Wu, B., Zhou, L., Chen, C., Wang, J., Hu, L. I., and Wang, X. (2022). Effects of exercise-induced hypoalgesia and its neural mechanisms. Med. Sci. Sports Exerc. 54, 220–231. doi: 10.1249/MSS.0000000000002781
Xiao, C. M., Li, J. J., Kang, Y., and Zhuang, Y. C. (2021). Follow-up of a Wuqinxi exercise at home programme to reduce pain and improve function for knee osteoarthritis in older people: a randomised controlled trial. Age Ageing 50, 570–575. doi: 10.1093/ageing/afaa179
Xiao, Z., and Li, G. (2021). The effect of exercises on the balance function and subjective quality of life in elderly, female knee osteoarthritis patients. Am. J. Transl. Res. 13, 6710–6716.
Xue, J.-F., Shi, Z.-M., Zou, J., and Li, X.-L. (2017). Inhibition of PI3K/AKT/mTOR signaling pathway promotes autophagy of articular chondrocytes and attenuates inflammatory response in rats with osteoarthritis. Biomed. Pharmacother. 89, 1252–1261. doi: 10.1016/j.biopha.2017.01.130
Yamaguchi, S., Aoyama, T., Ito, A., Nagai, M., Iijima, H., Zhang, X., et al. (2013). Effects of exercise level on biomarkers in a rat knee model of osteoarthritis. J. Orthop. Res. 31, 1026–1031. doi: 10.1002/jor.22332
Yang, J., Zhang, M., Yang, D., Ma, Y., Tang, Y., Xing, M., et al. (2021). mA-mediated upregulation of AC008 promotes osteoarthritis progression through the miR-328-3p-AQP1/ANKH axis. Exp. Mol. Med. 53, 1723–1734. doi: 10.1038/s12276-021-00696-7
Yang, Y., Wang, Y., Kong, Y., Zhang, X., and Bai, L. (2017). The effects of different frequency treadmill exercise on lipoxin A4 and articular cartilage degeneration in an experimental model of monosodium iodoacetate-induced osteoarthritis in rats. PLoS One 12:e0179162. doi: 10.1371/journal.pone.0179162
Yang, Y., Wang, Y., Kong, Y., Zhang, X., Zhang, H., Feng, X., et al. (2020). Moderate mechanical stimulation protects rats against osteoarthritis through the regulation of TRAIL via the NF-B/NLRP3 Pathway. Oxid. Med. Cell. Longev. 2020:6196398. doi: 10.1155/2020/6196398
Yang, Y., Wang, Y., Kong, Y., Zhang, X., Zhang, H., Gang, Y., et al. (2018). The therapeutic effects of lipoxin A during treadmill exercise on monosodium iodoacetate-induced osteoarthritis in rats. Mol. Immunol. 103, 35–45. doi: 10.1016/j.molimm.2018.08.027
Yang, Y., Wang, Y., Kong, Y., Zhang, X., Zhang, H., Gang, Y., et al. (2019). Mechanical stress protects against osteoarthritis via regulation of the AMPK/NF-κB signaling pathway. J. Cell. Physiol. 234, 9156–9167. doi: 10.1002/jcp.27592
Ye, D., Jian, W., Feng, J., and Liao, X. (2018). Role of long noncoding RNA ZFAS1 in proliferation, apoptosis and migration of chondrocytes in osteoarthritis. Biomed. Pharmacother. 104, 825–831. doi: 10.1016/j.biopha.2018.04.124
Yuenyongviwat, V., Duangmanee, S., Iamthanaporn, K., Tuntarattanapong, P., and Hongnaparak, T. (2020). Effect of hip abductor strengthening exercises in knee osteoarthritis: a randomized controlled trial. BMC Musculoskelet. Disord. 21:284. doi: 10.1186/s12891-020-03316-z
Yusup, A., Kaneko, H., Liu, L., Ning, L., Sadatsuki, R., Hada, S., et al. (2015). Bone marrow lesions, subchondral bone cysts and subchondral bone attrition are associated with histological synovitis in patients with end-stage knee osteoarthritis: a cross-sectional study. Osteoarthritis Cartilage 23, 1858–1864. doi: 10.1016/j.joca.2015.05.017
Zamli, Z., and Sharif, M. (2011). Chondrocyte apoptosis: a cause or consequence of osteoarthritis? Int. J. Rheum. Dis. 14, 159–166. doi: 10.1111/j.1756-185X.2011.01618.x
Zhang, H., Ji, L., Yang, Y., Wei, Y., Zhang, X., Gang, Y., et al. (2019). The therapeutic effects of treadmill exercise on osteoarthritis in rats by inhibiting the HDAC3/NF-KappaB Pathway and. Front. Physiol. 10:1060. doi: 10.3389/fphys.2019.01060
Zhang, X., Yang, Y., Li, X., Zhang, H., Gang, Y., and Bai, L. (2019). Alterations of autophagy in knee cartilage by treatment with treadmill exercise in a rat osteoarthritis model. Int. J. Mol. Med. 43, 336–344. doi: 10.3892/ijmm.2018.3948
Zhang, J., Qi, B., Liu, M., Zhao, T., and Tan, L. (2021). Influence of swimming exercise on the expression of apoptotic gene caspase-3 in chondrocytes in osteoarthritis. Am. J. Transl. Res. 13, 2511–2517.
Zhang, W., Qi, L., Chen, R., He, J., Liu, Z., Wang, W., et al. (2021). Circular RNAs in osteoarthritis: indispensable regulators and novel strategies in clinical implications. Arthritis Res. Ther. 23:23. doi: 10.1186/s13075-021-02420-2
Zhang, Z., Huang, L., Liu, Y., and Wang, L. (2020). Effect of Tai Chi training on plantar loads during walking in individuals with knee osteoarthritis. Biomed Res. Int. 2020:3096237. doi: 10.1155/2020/3096237
Zhang, W., He, L., Liu, Z., Ren, X., Qi, L., Wan, L., et al. (2020). Multifaceted functions and novel insight into the regulatory Role of RNA N-methyladenosine modification in musculoskeletal disorders. Front. Cell Dev. Biol. 8:870. doi: 10.3389/fcell.2020.00870
Zheng, K., Chen, C., Yang, S., and Wang, X. (2021). Aerobic exercise attenuates pain sensitivity: an event-related potential study. Front. Neurosci. 15:735470. doi: 10.3389/fnins.2021.735470
Zhou, X., Cao, H., Wang, M., Zou, J., and Wu, W. (2021). Moderate-intensity treadmill running relieves motion-induced post-traumatic osteoarthritis mice by up-regulating the expression of lncRNA H19. Biomed. Eng. Online 20:111. doi: 10.1186/s12938-021-00949-6
Zhu, J., Yu, W., Wang, Y., Xia, K., Huang, Y., Xu, A., et al. (2019). lncRNAs: function and mechanism in cartilage development, degeneration, and regeneration. Stem Cell Res. Ther. 10:344. doi: 10.1186/s13287-019-1458-8
Keywords : osteoarthritis, exercise, pathology, mechanism, therapy
Citation: Kong H, Wang X-Q and Zhang X-A (2022) Exercise for Osteoarthritis: A Literature Review of Pathology and Mechanism. Front. Aging Neurosci. 14:854026. doi: 10.3389/fnagi.2022.854026
Received: 13 January 2022; Accepted: 11 March 2022; Published: 03 May 2022.
Reviewed by:
Copyright © 2022 Kong, Wang and Zhang. This is an open-access article distributed under the terms of the Creative Commons Attribution License (CC BY) . The use, distribution or reproduction in other forums is permitted, provided the original author(s) and the copyright owner(s) are credited and that the original publication in this journal is cited, in accordance with accepted academic practice. No use, distribution or reproduction is permitted which does not comply with these terms.
*Correspondence: Xin-An Zhang, [email protected] ; Xue-Qiang Wang, [email protected]
This article is part of the Research Topic
Physical Exercise for Age-Related Neuromusculoskeletal Disorders
- Systematic review update
- Open access
- Published: 19 March 2024
The effects of manual therapy in pain and safety of patients with knee osteoarthritis: a systematic review and meta-analysis
- Bowen Zhu 1 na1 ,
- He Ba 2 na1 ,
- Lingjun Kong 1 ,
- Yangyang Fu 3 ,
- Jun Ren 1 ,
- Qingguang Zhu 3 , 4 &
- Min Fang 1 , 4
Systematic Reviews volume 13 , Article number: 91 ( 2024 ) Cite this article
26 Accesses
22 Altmetric
Metrics details
Manual therapy (MT) is frequently used in combination with management of osteoarthritis of the knee, but there is no consensus on the exact efficacy of this treatment strategy. The purpose of this systematic review and meta-analysis was to evaluate the pain relief and safety of MT for treatment of knee osteoarthritis (KOA).
Randomized controlled trials evaluating MT in patients with KOA in major English and Chinese journals were searched in the following databases: Wanfang, China Science and Technology Journal Database (VIP database), China National Knowledge Infrastructure (CNKI), PubMed, Embase, Web of Science, and the Cochrane Library databases through June 2023. The methodological quality and quality of evidence of the included studies were assessed using Cochrane’s risk-of-bias 2 (ROB 2) tool and the Grading of Recommendations Assessment, Development, and Evaluation (GRADE) tool. Data analysis was performed using Stata version 15.0 software. After use of Galbraith plots to exclude studies that could lead to heterogeneity, random effects models were used to analyze the remaining data and test the consistency of the findings. We used meta-regression to assess the effect of treatment period, patient age, and sex ratio on outcomes. Funnel plots and Egger’s test were used to evaluate publication bias. Sensitivity analyses were used to determine the reliability of the results.
A total of 25 studies, with 2376 participants, were included in this review. The overall methodological quality of the included studies was limited. Our findings suggest that MT has a positive impact on pain relief outcomes in KOA patients. The meta-analysis showed that MT was superior to usual care ( SMD = 2.04, 95% CI 0.94, 3.14, I 2 = 96.3%; low evidence quality) and exercise ( SMD = 1.56, 95% CI 0.41, 2.71, I 2 = 96.3%; low evidence quality) for reducing pain. In terms of improvement in visual analogue scale (VAS) scores, MT treatment beyond 4 weeks ( SMD = 1.56, 95% CI 0.41, 2.71, I 2 = 96.3%) may be superior to treatments less than or equal to 4 weeks ( SMD = 1.24, 95% CI 0.56, 1.95, I 2 = 94.7%). No serious adverse events associated with MT were reported.
Conclusions
MT may be effective at reducing pain in patients with KOA and may be more effective after a 4-week treatment period. Compared with usual care and exercise therapy, MT may be superior at reducing KOA pain in the short term (9 weeks), but its long-term efficacy requires careful consideration of evidence-based outcomes. MT appears to be safe for KOA patients, though clinicians should inform patients of the potential risk of MT-related adverse events.
Peer Review reports
Knee osteoarthritis (KOA) is a chronic degenerative disease of the knee joint, and knee joint pain is the most common clinical manifestation [ 1 ]. The worldwide prevalence of radiographically confirmed symptomatic KOA is estimated to be 3.8%, and the prevalence of this disease has increased to more than 10% in people older than 60 years [ 2 ]. In China, the prevalence of KOA among older people is approximately 8.5% [ 3 ], and the incidence of KOA has increased significantly among younger people [ 4 ]. KOA has a serious impact on the health status of patients and overall quality of life and can even cause a serious economic burden on society [ 5 ]. Clinical guidelines recommend that relieving pain in the knee is a primary target of KOA treatment [ 6 , 7 ].
Manual therapy (MT), including massage therapy and manipulative therapy, is a widely used conservative treatment strategy [ 8 ]. MT may have a positive effect on reducing pain [ 9 , 10 ] and is reportedly used by approximately 15.4 million people in the USA for treatment of KOA, for example [ 11 ]. In some countries, MT is considered a first-line treatment option [ 12 ], whereas in others, it is recommended to be used as part of a broader treatment program that includes exercise; alternatively, MT is not recommended because of a lack of evidence [ 13 ]. Despite widespread use of MT, few studies have reported the efficacy of MT alone for treatment of KOA, with poor methodological quality [ 14 ]. Due to the lack of evidence, there is no consensus about recommending MT for KOA patients.
In recent years, several randomized controlled clinical trials (RCTs) have been conducted to assess the efficacy of manipulation in management of KOA, particularly in China. Indeed, Chinese massage therapy, including soft tissue manipulation and joint manipulation, has been used by practitioners for management of KOA, and trials have shown good results in terms of reducing pain [ 15 , 16 ]. Therefore, additional evidence-based evidence is needed to explore the analgesic efficacy of MT.
This study was conducted by performing a systematic review and meta-analysis to assess the benefits of MT alone in management of KOA, mainly in terms of improving pain and updating the effects of MT on patients with KOA, to provide empirical evidence and reference for clinical application of MT in treatment of KOA.
The protocol was registered after all phases of the review process were completed, and the manuscript was finished. This study was performed strictly by following Preferred Reporting Items for Systematic Reviews and Meta-Analyses (PRISMA) statement guidelines [ 17 ]. The INPLASY registration number was INPLASY 202360030.
Literature search
We conducted a literature search of the following databases for articles published from inception to December 2021 with the language restricted to English or Chinese, with an updated search conducted in July 2023: Wanfang Database, China Science and Technology Journal Database (VIP database), China National Knowledge Infrastructure (CNKI), PubMed, Embase, Web of Science, and the Cochrane Library databases. All keywords were mapped to “indexed items” (e.g., MeSH) using a combination of the following in Embase.
#1'knee'/exp.
#2knee*:ti,ab,kw.
#3[< 1966–2023]/py.
#4 (#1 OR #2) AND #3
#5'arthritis'/exp OR 'osteoarthritis'/exp.
#6arthrit*:ti,ab,kw OR osteoarthr*:ti,ab,kw.
#7[< 1966–2023]/py.
#8 (#5 OR #6) AND #7
#9'massage'/exp OR 'musculoskeletal manipulation'/exp.
#10massage*:ti,ab,kw OR 'zone therap*':ti,ab,kw OR manipul*:ti,ab,kw.
#11[< 1966–2023]/py.
#12 (#9 OR #10) AND #11
#13'western ontario and mcmaster universities osteoarthritis index'/exp OR 'visual analog scale'/exp.
#14'western ontario and mcmaster universities osteoarthritis index*' OR womac.
#15'visual analog scale' OR vas.
#16 #13 OR #14 OR #15
#17'randomized controlled trial'/exp OR 'randomized controlled trial (topic)'/exp OR 'controlled clinical trial'/exp OR 'randomization'/exp OR 'double blind procedure'/exp OR 'single blind procedure'/exp.
#18 (clinic* NEAR/2 trial*):ti,ab,kw.
#19random*:ti,ab,kw OR placebo*:ti,ab,kw OR blind*:ti,ab,kw OR mask*:ti,ab,kw.
#20 #17 OR (#18 AND #19)
#4 AND #8 AND #12 AND #16 AND #20
The detailed search strategy is shown as an example in Appendix 1 .
Study selection
Only RCTs that reported the method of randomization to MT alone for KOA were included. In the case of a three-arm or multiarm RCT, articles were included if two of the groups met the inclusion criteria [ 18 ]. All patients with KOA were included, regardless of age, race, sex, age limit, or severity. If a study did not report information on the randomization method, ethics approval, or clinical study registration, it was excluded. Case reports, empirical reports, and laboratory studies were not included.
Eligibility criteria
Patients included in the study had a clear diagnosis of KOA and met other diagnostic criteria, such as the American College of Rheumatology criteria or the Chinese Medical Association Orthopaedic Branch Guidelines for the Treatment of Osteoarthritis (2018), with no restrictions on the severity of the disease. Interventions in the experimental group involved only MT, and those in the control group involved any therapy other than MT (such as acupuncture treatment, medication, exercise, and usual care). In addition, if the observed differences were thought to be due to the unique contribution of MT, we included studies that may encompass research in which MT was provided as part of a package of care, that is, if the effects of MT could be isolated. For example, studies comparing MT plus usual care with usual care alone were included, whereas investigations comparing MT plus usual care with MT alone were not. We also excluded studies in which MT was combined with other therapies because it was difficult to distinguish the effect of MT.
Outcome analyses
The effect of MT in combination with any other therapeutic adjuncts (including usual care, herbal application, oral analgesics, exercise, acupuncture) was examined. The primary outcome was the VAS pain assessment scale [ 19 ]. Secondary outcomes were the Western Ontario and McMaster Universities Osteoarthritis Index (WOMAC) pain scale [ 20 ], follow-up data, and adverse events.
Data extraction
Two authors (B. Z. and H. B.) independently collected the data, including the following: number of subjects, sex, age, body mass index (BMI), duration of disease, country, MT intervention period, diagnostic criteria for KOA, Kellgren–Lawrence grade, VAS score, WOMAC pain score, follow-up duration, and incidence of adverse events. In the meta-analysis, VAS and/or WOMAC pain scores were extracted. In the absence of sufficient data, the corresponding authors were contacted for additional data.
Risk-of-bias assessment
Risk of bias in RCTs was assessed according to the revised Cochrane risk of bias in randomized trials tool (RoB2) [ 21 ]. All risk-of-bias assessments were conducted in duplicate by 2 reviewers (L. K. and R. J.). Disagreements were resolved by recruiting a third author (Y. F.) to reach a consensus.
Quality of the evidence: Cochrane GRADE assessment
The Grading of Recommendations, Assessment, Development and Evaluation (GRADE) methodology [ 22 ] is a system for grading the quality of evidence for health recommendations and assessing the quality of evidence [ 23 ]. The quality of the evidence is rated from “very low” to “high”: (a) high (the true effect is considered close to the estimated effect), (b) moderate (confidence in the estimated effect is moderate), (c) low (confidence in the effect is limited), and (d) very low (confidence in the effect is very limited, and there is a high degree of uncertainty about the outcome) [ 24 ]. Scores were reviewed by the senior author (L. K.).
Data synthesis and analysis
All the search results were imported into NoteExpress v3.5.0.9054 for management. Two reviewers independently screened all potentially eligible studies. Titles and abstracts were screened first to exclude irrelevant citations. The full texts of all articles with potentially relevant abstracts were retrieved and screened according to the study eligibility criteria. Disagreements were resolved by consensus or discussion with a third reviewer.
The primary endpoint of this meta-analysis was the difference in VAS score between patients receiving MT and those receiving other therapies. Adverse events are summarized narratively. All pain scales were converted to a 10-point scale. The secondary endpoint was the difference in WOMAC pain scores (0–20) between these two groups of patients. The negative effect size of the VAS score or WOMAC score indicates that MT was more beneficial than other therapies, indicating that the participants had less pain. Primary and secondary outcomes were collected, and the data were analyzed after randomization and at the end of treatment. Minimum clinically important difference (MCID) thresholds for the VAS and WOMAC scores were defined as a 20% fluctuation from the baseline of the included studies based on previous studies and were calculated as follows: 1.18/10 for the VAS for pain and 2.12/20 for the WOMAC pain score [ 25 ].
We extracted final value scores (means and standard deviations) for the meta-analysis and converted change scores into mean values [ 26 , 27 ]. Heterogeneity among the included studies was assessed using the Q -test and is presented as I 2 and P -values. An I 2 > 50% and/or a P -value < 0.1 indicated significant heterogeneity among the studies. If the heterogeneity test showed significant heterogeneity, a random effects model was used; otherwise, a fixed effects model was applied [ 28 ]. To explore the source of heterogeneity, we conducted subgroup analysis of VAS scores based on differences in treatment methods. The subgroup analysis of VAS scores was based on the course of treatment (set cut-off value of 4 weeks); although we considered the effect of the course of MT because it was not included in our previously designed protocol, we included this comparison because it represents a point for continuing discussion. We also used the Galbraith plot to explore studies that may have contributed to the heterogeneity and excluded them.
Then, we used a random effects model to analyze the remaining data, and we observed that the results were consistent with those obtained previously [ 29 ]. Meta-regression was used to evaluate the effect of the treatment period and sex ratio on the results, and the threshold for statistical significance was set at P < 0.05. We used a funnel plot and Egger’s test to evaluate publication bias ( P < 0.1 was considered to indicate significant publication bias among the enrolled studies). To assess the reliability of the findings in this study, sensitivity analyses were also conducted. All analyses were carried out with Stata version 15.0 (Stata Corporation, College Station, TX, USA).
There were no public or patient representatives directly involved in the drafting or process of this review.
Search and selection
The literature search identified 6990 records, and a total of 3184 potential studies were identified after removing duplicates. After screening the titles and abstracts, 3072 studies were excluded, and the remaining 119 full texts were screened for inclusion. After the detailed full-text screening, 39 studies were included in the qualitative synthesis. Finally, 25 RCTs were included in the current review [ 30 , 31 , 32 , 33 , 34 , 35 , 36 , 37 , 38 , 39 , 40 , 41 , 42 , 43 , 44 , 45 , 46 , 47 , 48 , 49 , 50 , 51 , 52 , 53 , 54 ]. The detailed process of the search and selection is shown in Fig. 1 .
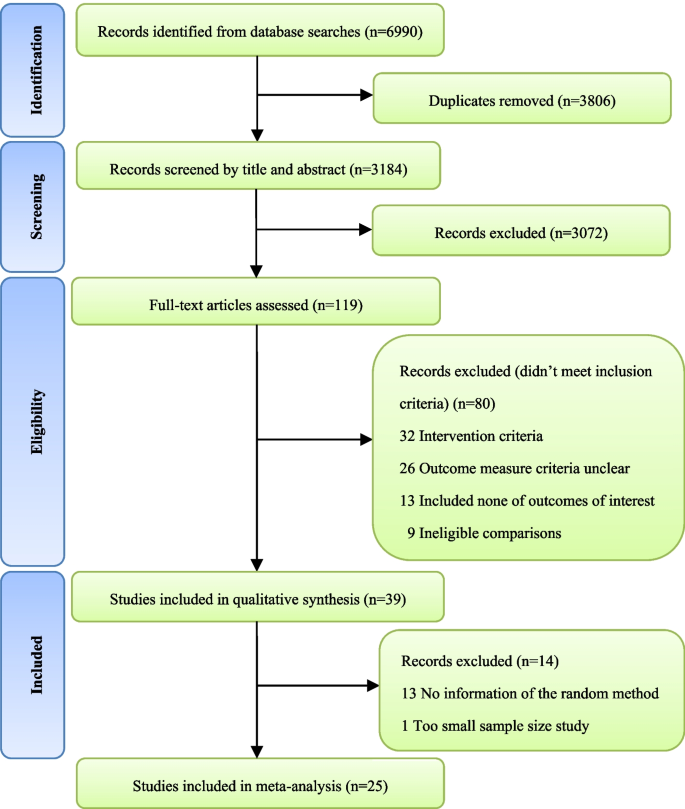
Selection of studies through review
Risk-of-bias assessment of pain outcomes
Three of the 25 included studies were considered to have a moderate risk of bias [ 22 , 30 , 44 ]; the remaining 22 studies all showed a high risk of bias. The randomization process revealed some concern, with a high risk of bias. The common areas of bias were selection bias (allocation concealment) and performance bias (blinding of participants and/or healthcare providers). All the included studies failed to meet at least one of the two criteria. The detailed risk-of-bias assessment of pain outcomes is shown in Fig. 2 and Appendix 2 .
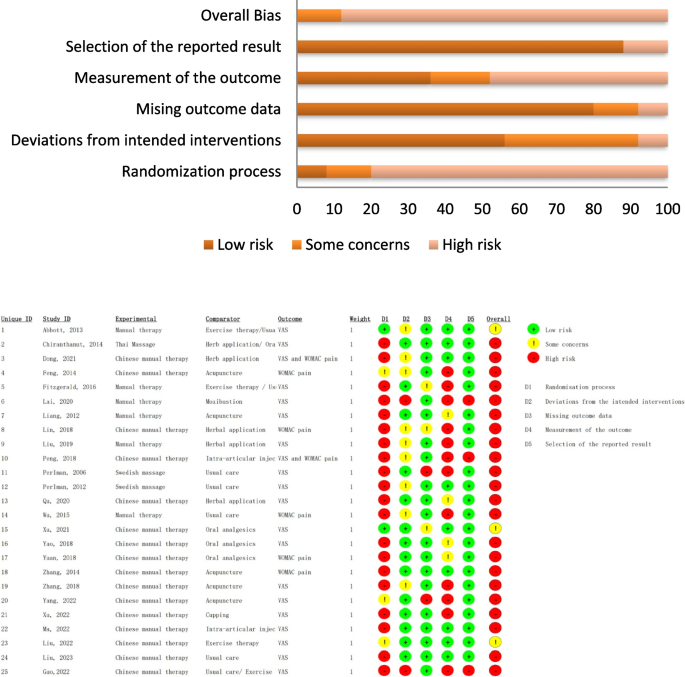
Results of the risk-of-bias assessment using RoB2
Effects of interventions
The treatment effects, quality of the evidence, and GRADE summary of VAS scores for all comparisons among the included trials are summarized in Table 1 . The GRADE approach to evidence synthesis and operationalization of criteria items are shown in Appendix 3 .
MT compared with other therapies in VAS score of KOA patients
The included studies and population.
Twenty trials (25 cohorts) published between 2013 and 2023 included 2376 patients (mean age was 61.38 years) and reported changes in the VAS scores of KOA patients who received MT or other therapies. Eleven trials [ 31 , 32 , 36 , 38 , 44 , 45 , 49 , 50 , 51 , 52 , 53 ] reported the severity of the disease in patients using the Kellgren-Lawrence criteria, and the remaining studies included patients with severity levels of III or less, except for two trials [ 31 , 38 ] that were graded IV. Five trials were published in English and 15 in Chinese. A detailed description of the characteristics of the studies is available upon request from the primary author. The sample sizes of the studies ranged from 40 to 448. The detailed characteristics of each included study are listed in Table 2 .
Patients treated with MT had a significantly greater VAS score than did those who received other therapies (standardized mean difference (SMD) 0.68, 95% CI : 0.31 to 1.06), with a significantly greater effect size ( Z = 3.56, P < 0.01); however, the effect was considered small. Significant heterogeneity was found among the enrolled studies ( I 2 = 94.4%, P = 0.000) (Fig. 3 ).
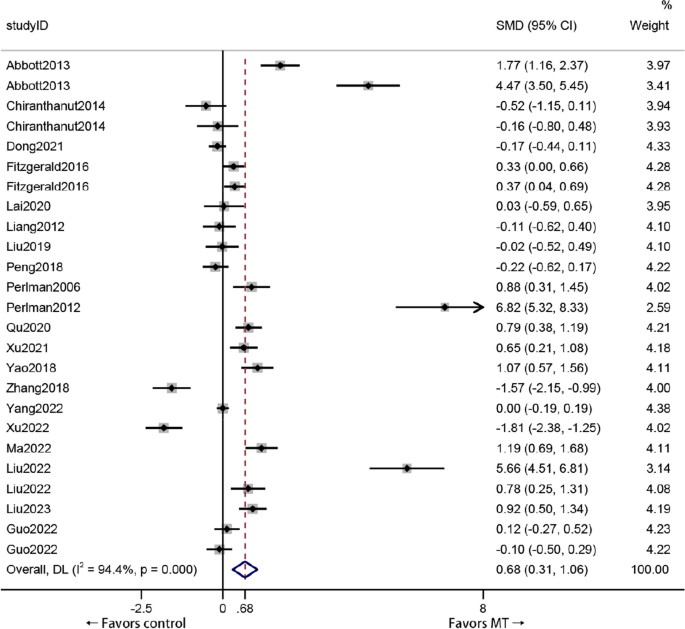
Forest plot comparing the VAS score reductions of KOA patients who received MT and other treatments
Compared with other therapies
The results of subgroup analysis based on the type of intervention showed that compared with those in the usual care group, improvements in the VAS score were more significant in the MT group ( P = 0.000). Moreover, improvements in the VAS score in the MT group were significant ( P = 0.008). However, compared with that in the herbal application, oral analgesic, acupuncture, and intra-articular injection groups, the VAS score in the MT group showed no significant improvement ( P > 0.05) at the end of intervention (Fig. 4 ).
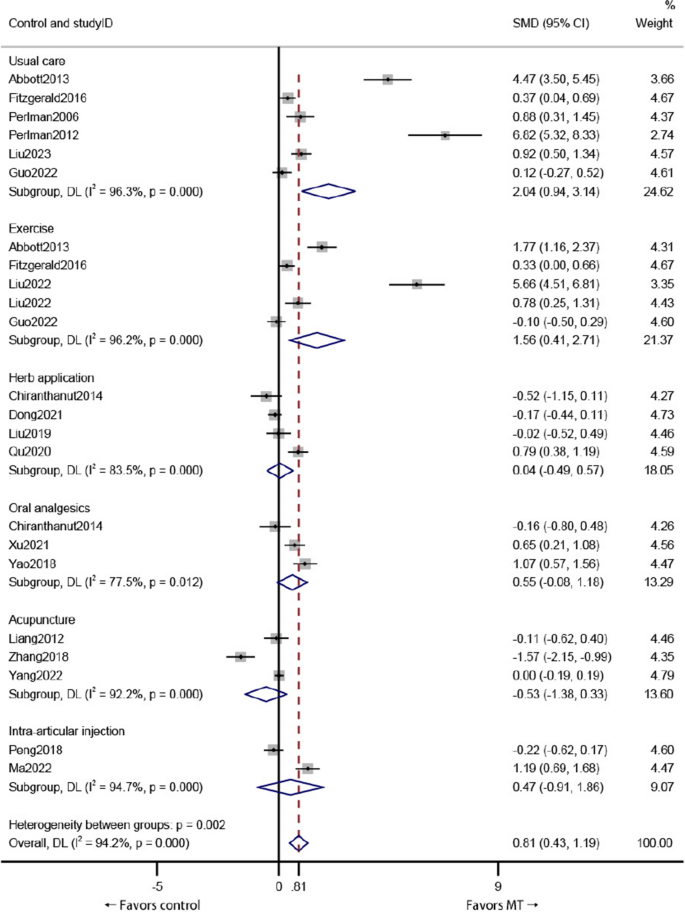
Subgroup analysis of VAS score outcomes based on the type of intervention in the control group
Compared with other interventions, MT treatments of more than 4 weeks ( P = 0.000) may be superior to treatments of less than or equal to 4 weeks ( P = 0.136) in terms of improvement in VAS score (Fig. 5 ).
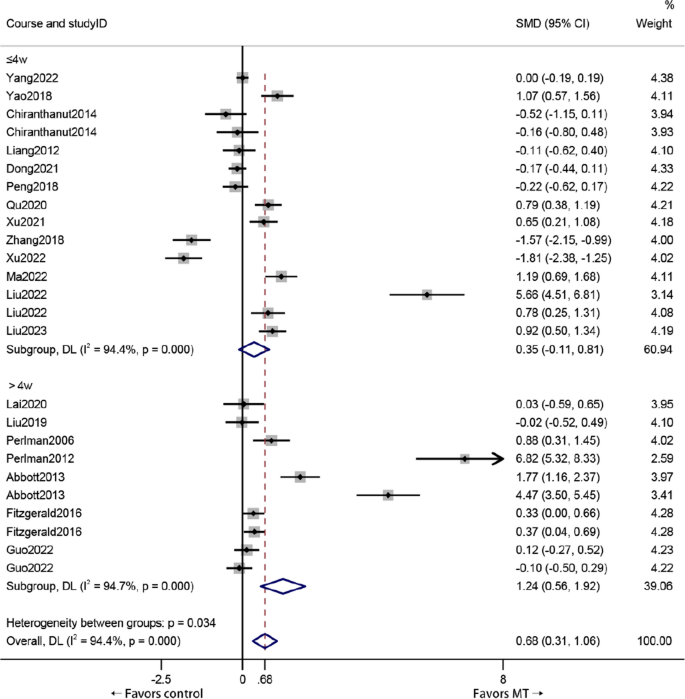
Subgroup analysis of VAS score outcomes based on course of treatment
Secondary outcome
The effect of mt compared with other therapies on the womac pain score in koa patients.
Eight trials (9 cohorts) that included 877 patients reported changes in the WOMAC pain scores of KOA patients who received MT or other therapies. As shown in Fig. 6 , compared with patients who received other therapies, patients treated with MT did not show significant changes in WOMAC pain scores (standardized mean difference (SMD) 0.27, 95% CI : − 0.07 to 0.61), with a small effect size ( Z = 1.561, P = 0.119). Significant heterogeneity was found among the studies ( I 2 = 83.5%, P = 0.000). MT failed to improve the WOMAC pain score of KOA patients compared with that of patients receiving other therapies.
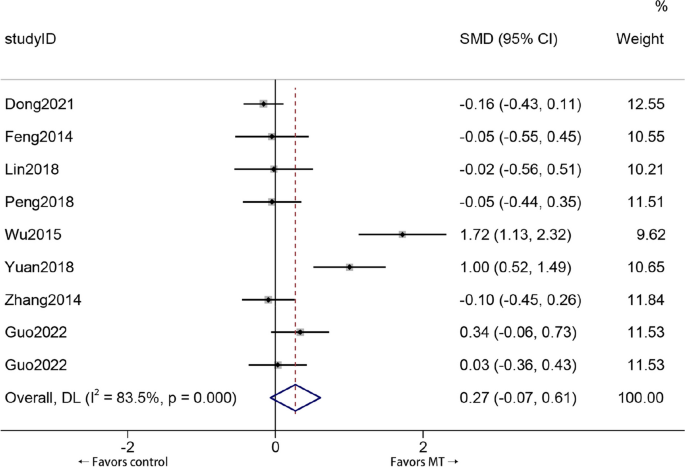
Forest plot comparing the WOMAC pain score reductions of KOA patients who received MT and other treatments
Follow-up and treatment cycle
The included studies ranged from 1.5 to 9 weeks of treatment for MT. Eight studies [ 30 , 32 , 34 , 37 , 39 , 40 , 41 , 54 ] reported follow-up results, with a minimum follow-up of 1 month and a maximum follow-up of 1 year, as shown in Table 3 . In terms of pain relief, 6 [ 30 , 32 , 37 , 39 , 40 , 41 ] of these follow-ups found moderate to satisfactory long-term effects of MT in the treatment of KOA, 1 [ 34 ] study showed only short-term benefits for MT, and 1 [ 54 ] study did not report follow-up statistics.
Adverse reactions
Fourteen studies reported adverse events [ 30 , 31 , 32 , 34 , 37 , 38 , 40 , 41 , 44 , 49 , 50 , 51 , 52 , 53 ]. Muscle soreness was the most common side effect of MT after treatment. Mild aggravation of pain occurred in one patient [ 44 ]. In most studies, adverse events were not reported.
Galbraith plot for analysis of heterogeneity across studies
Galbraith plots are an alternative to the forest plots proposed by Galbraith for visualizing the results of studies and meta-analyses, and Galbraith plots also aid in detecting sources of heterogeneity [ 55 , 56 ]. Two lines are drawn at a vertical distance of ± 2 above and below the regression line, and these two lines together with the regression line constitute an interval. According to the relevant literature, studies that were published completely outside this interval may be responsible for the observed heterogeneity [ 33 ]. Therefore, we excluded all 15 studies outside the interval and performed a secondary analysis on the remaining 10 studies (Fig. 7 ). As shown in Fig. 8 , the secondary analysis reached the same conclusion as before: compared with other treatments, MT was more effective at improving the VAS score of KOA patients (standardized mean difference (SMD) = 0.23, 95% CI = 0.009 to 0.36, Z = 3.233, P = 0.001). Moreover, the heterogeneity between studies was reduced ( I 2 = 44.7%, P = 0.061).
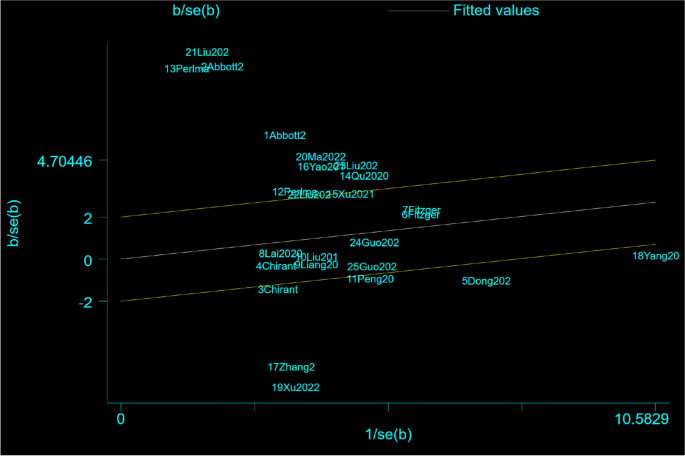
Using Galbraith plot to infer studies that might be the source of heterogeneity
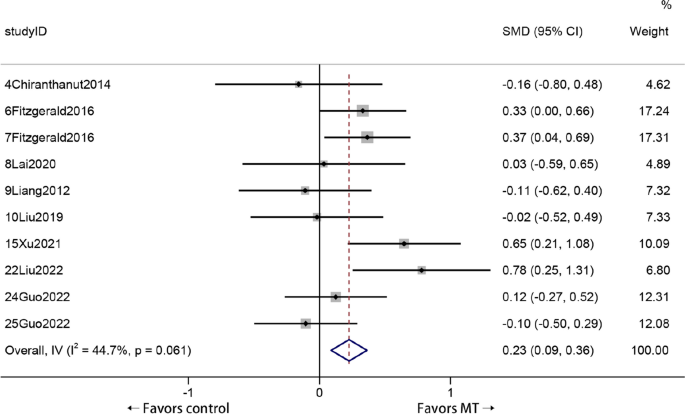
Comparing the VAS score reductions of KOA patients who received MT and other treatments with the remaining studies
Publication bias and sensitivity analysis
In this meta-analysis, we assessed publication bias using funnel plots and Egger’s test [ 57 ], and our results indicated no significant publication bias ( P egger = 0.442) in the included studies (Appendix 4 ). We examined the effect of classifying MT with other therapies in sensitivity analysis, which did not affect our results. Sensitivity analysis indicated that the results were stable (Appendix 5 ).
Meta-regression
As differences in the treatment period, patient age, and sex ratio may have affected the analysis results, we performed meta-regression to evaluate whether the above factors had a significant impact on the results. Overall, the treatment duration, mean age of patients, and sex ratio did not significantly affect the results (Appendix 6 ).
This study constitutes an update of a comprehensive systematic review to assess the efficacy of MT in conservative management of patients with KOA for pain relief, with more stringent inclusion criteria to ensure the quality of the source RCTs. Considerable effort was made to conduct an extensive literature search. Twenty of the 25 studies were conducted in China, 3 in the USA, and 1 each in New Zealand and Thailand. Most of the included studies used MT.
This study integrated evidence from existing clinical RCTs of MT for KOA pain and identified proposed research targets that remain to be addressed. Our findings on VAS score improvement in patients with KOA were statistically significant but may not be clinically meaningful when compared to those for patients receiving other therapies in addition to usual care and exercise. Although no significant differences in WOMAC pain scores were found for the secondary outcomes, MT appears to have the potential and is undeniably effective at reducing pain in KOA patients. In this meta-analysis, we used the MCID as a threshold to assess the clinical significance of the difference between the MT group and the control group rather than relying solely on statistical significance. There are anchor-based and distribution-based methods for calculating the MCID, and based on previous studies, we set the threshold at 20% using the anchor-based method [ 58 , 59 , 60 ]. According to our subgroup analyses, the effect of MT on the VAS score at the end of the intervention exceeded the effect of the MCID in both the usual care and exercise groups but not in other control groups. These findings suggest that MT may have better clinical applicability in the short term than usual care and exercise. The findings, including the Chinese database search, support previous meta-analyses in the literature [ 61 ]. Although guidelines [ 62 ] report that exercise treatment might be more effective than MT at reducing pain intensity at short-term follow-up, our meta-analysis showed that MT was no less effective than exercise interventions for pain relief in patients with KOA and was superior to usual care. Nevertheless, because most of the studies included in this systematic review were carried out in China, the results may be influenced to some extent by the limitations of factors such as geographic location and cultural group, and additional dimensions of data should be added in the future to assess the stability of the findings.
In terms of outcome indicators, MT did not significantly differ with regard to WOMAC pain score, indicating that MT has limitations in relieving pain and does not cover the full range of patients’ daily lives, especially for those in pain situations such as walking, moving up and downstairs, sleeping, standing, sitting, and lying down. However, the VAS for global pain had a slightly greater assay sensitivity at the trial and meta-analysis levels than the WOMAC pain subscale [ 63 ]. In contrast to comprehensive assessment of the efficacy of the VAS, the WOMAC pain score consists of five questions, and the results are inherently multidimensional; e.g., the pain relief effect of MT might be limited in some specific activities, resulting in biased scores, but this needs to be validated by additional studies [ 64 ]. Furthermore, RCTs of MT for KOA treatment are still rare because of the lack of research on long-term treatment in particular, and additional RCTs on the effects of MT for KOA treatment are needed, as are studies integrating MT with biomechanics.
MT appears to be safe for individuals with KOA. In our review, 56% of the included studies reported safety information, with few adverse events described and no serious adverse events shown. A meta-analysis of adverse events of MT in RCTs found that most of the observed adverse events were musculoskeletal related, transient in nature, or mild to moderate in severity [ 65 ]. Our review also supports this conclusion by revealing that muscle discomfort and pain caused by MT were the most common adverse events. However, most existing meta-analyses on MT in KOA patients do not include safety/adverse effects because poor reporting of safety information in MT clinical trials is common in other populations. We strongly suggest that future investigators monitor and report safety/adverse events of MT in clinical trials for KOA, as recommended by CONSORT.
We could better explain the effects if more attention was given to the qualitative components of the intervention, such as the context of the visit, patient beliefs, and preferences. Although we focused on the impact of MT on the level of pain in KOA patients in this review, the economic costs associated with care and psychophysiological mechanisms should also be considered. In particular, this approach has the following advantages: changes in parasympathetic activity (as measured by heart rate, blood pressure, and heart rate variability) and hormonal levels (as measured by cortisol levels) following MT result in a relaxation response (physiological mechanisms); a reduction in anxiety and an improvement in mood state after MT cause relaxation (psychological mechanisms) [ 66 ]. The treatment cycle of MT is typically 1.5 to 9 weeks, and follow-up results show that MT is more effective for pain relief in the short term and appears to have improved efficacy in the long term, which may be maintained for up to 1 year; however, the outcome may not be completely stable or reliable. One study [ 34 ] indicated that MT results were unsatisfactory at follow-up to 1 year. This may also be limited by the short treatment period of MT, and the effectiveness and long-term efficacy of interventions with long MT sessions may be worth exploring. Furthermore, the most recent systematic review [ 67 ] on the cost-effectiveness of noninvasive and nonpharmacologic interventions for KOA concluded that studies of other economic assessments of MT for KOA are rare. For example, with other conservative treatment options, assessing whether a long course of MT treatment (> 4 weeks) provides the best economic benefit, and therefore, whether MT is a cost-effective option for treating KOA remains to be determined.
Limitations
First, because of the different settings and populations (age, occupation, and socioeconomic status) in the studies and use of different recruitment methods and MT techniques, we could not perfectly address the issues related to statistical heterogeneity. Although we implemented subgroup analyses of different techniques (results not shown) and validated the results using Galbraith plots, such results will be difficult to address in future reviews. Second, some studies were excluded, even though WOMAC pain scores were collected. These studies did not report methods for evaluating WOMAC pain or matching our inclusion criteria, which made it difficult to integrate the WOMAC pain data. Finally, most studies ignore classifying the severity of KOA unreported in the Kellgren–Lawrence grading system. Hence, the inconsistency of the severity of the disease limits the number of studies, and we were unable to conduct subgroup analysis according to the severity of the disease to explore its effect in more depth.
Implications
The findings suggest that MT as a stand-alone treatment may not produce satisfactory meaningful pain relief, especially in some elderly KOA patients who present with pain only in certain circumstances. The method of adverse event assessment was unclear. In general, MT may result in a temporary mild increase in muscle soreness but is essentially safe. Therefore, if a patient chooses nonsurgical conservative treatment, clinicians treating KOA patients may safely prioritize MT in anticipation of a possible short-term improvement in pain.
MT is potentially effective at reducing pain in KOA patients, and long-term treatment periods may be more effective. While there may be limitations to the effectiveness of MT, it may be more advantageous than usual care and exercise therapy, but the results need to be referenced with caution. MT seems to be safe in patients with KOA, but better monitoring and reporting of security information are strongly recommended. Overall, there is a lack of large samples of randomized controlled trials and active research evaluating the economic benefits of MT. More high-quality studies are needed in the future to determine the beneficial effects of MT on pain in patients with KOA.
Availability of data and materials
The datasets supporting the conclusions of this article are included within the article.
Hunter DJ, Bierma-Zeinstra S. Osteoarthritis Lancet. 2019;393:1745–59.
CAS PubMed Google Scholar
Brophy RH, Fillingham YA. AAOS clinical practice guideline summary: management of osteoarthritis of the knee (nonarthroplasty), third edition. J Am Acad Orthop Surg. 2022;30:e721–9.
Article PubMed Google Scholar
Ren Y, Hu J, Tan J, Tang X, Li Q, Yang H, et al. Incidence and risk factors of symptomatic knee osteoarthritis among the Chinese population: analysis from a nationwide longitudinal study. BMC Public Health. 2020;20:1491.
Article PubMed PubMed Central Google Scholar
Chen H, Wu J, Wang Z, Wu Y, Wu T, Wu Y, et al. Trends and patterns of knee osteoarthritis in China: a longitudinal study of 17.7 million adults from 2008 to 2017. Int J Environ Res Public Health. 2021;18:8864.
Wojcieszek A, Kurowska A, Majda A, Liszka H, Gądek A. The impact of chronic pain, stiffness and difficulties in performing daily activities on the quality of life of older patients with knee osteoarthritis. Int J Environ Res Public Health. 2022;19:16815.
Arden NK, Perry TA, Bannuru RR, Bruyère O, Cooper C, Haugen IK, et al. Non-surgical management of knee osteoarthritis: comparison of ESCEO and OARSI 2019 guidelines. Nat Rev Rheumatol. 2021;17:59–66.
Article CAS PubMed Google Scholar
NICE. Osteoarthritis: care and management. London: National Institute for Health and Care Excellence (NICE); 2020.
Yong RJ, Mullins PM, Bhattacharyya N. Prevalence of chronic pain among adults in the United States. Pain. 2022;163:e328–32.
Bervoets DC, Luijsterburg PA, Alessie JJ, Buijs MJ, Verhagen AP. Massage therapy has short-term benefits for people with common musculoskeletal disorders compared to no treatment: a systematic review. J Physiother. 2015;61:106–16.
Pehlivan S, Karadakovan A. Effects of aromatherapy massage on pain, functional state, and quality of life in an elderly individual with knee osteoarthritis. Jpn J Nurs Sci. 2019;16:450–8.
Clarke TC, Black LI, Stussman BJ, Barnes PM, Nahin RL. Trends in the use of complementary health approaches among adults: United States, 2002–2012. Natl Health Stat Report. 2015;79:1–16.
Google Scholar
Zhang Z, Huang C, Jiang Q, Zheng Y, Liu Y, Liu S, et al. Guidelines for the diagnosis and treatment of osteoarthritis in China (2019 edition). Ann Transl Med. 2020;8:1213.
Krishnamurthy A, Lang AE, Pangarkar S, Edison J, Cody J, Sall J. Synopsis of the 2020 US Department of Veterans Affairs/US Department of Defense clinical practice guideline: the non-surgical management of hip and knee osteoarthritis. Mayo Clin Proc. 2021;96:2435–47.
Xu Q, Chen B, Wang Y, Wang X, Han D, Ding D, et al. The effectiveness of manual therapy for relieving pain, stiffness, and dysfunction in knee osteoarthritis: a systematic review and meta-analysis. Pain Physician. 2017;20:229–43.
PubMed Google Scholar
Zhang Z. A self-control study based on MRI to evaluate the influence of massage manipulation on cartilage metabolism in patients with knee osteoarthritis. China Acad Chin Med Sci. 2020;66:1–4.
Nasiri A, Mahmodi MA. Aromatherapy massage with lavender essential oil and the prevention of disability in ADL in patients with osteoarthritis of the knee: a randomized controlled clinical trial. Complement Ther Clin Pract. 2018;30:116–21.
Shamseer L, Moher D, Clarke M, et al. Preferred Reporting Items for Systematic Review And Meta-Analysis Protocols (PRISMA-P) 2015: elaboration and explanation. BMJ. 2015;350: g7647.
Higgins JPT, Green S. Cochrane Handbook for Systematic Reviews of Interventions. London: The Cochrane Collaboration; 2011.
Anagnostis C, Mayer TG, Gatchel RJ, Proctor TJ. The million visual analog scale: its utility for predicting tertiary rehabilitation outcomes. Spine (Phila Pa 1976). 2003;28:1051–60.
Bellamy N, Buchanan WW, Goldsmith CH, Campbell J, Stitt LW. Validation study of WOMAC: a health status instrument for measuring clinically important patient relevant outcomes to antirheumatic drug therapy in patients with osteoarthritis of the hip or knee. J Rheumatol. 1988;15:1833–40.
Sterne JAC, Savović J, Page MJ, Elbers RG, Blencowe NS, Boutron I, et al. RoB 2: a revised tool for assessing risk of bias in randomised trials. BMJ. 2019;366: l4898.
Ministry of Health. Secretariat for science, technology and strategic input. Methodological guidelines: development of systematic review and meta-analysis of randomized clinical trials. Brasília: Ministé-rio da Saúde; 2021.
Atkins D, Eccles M, Flottorp S, Guyatt GH, Henry D, Hill S, et al. Systems for grading the quality of evidence and the strength of recommendations I: critical appraisal of existing approaches the GRADE working group. BMC Health Serv Res. 2004;4:38.
Balshem H, Helfand M, Schünemann HJ, Oxman AD, Kunz R, Brozek J, et al. GRADE guidelines: 3. Rating the quality of evidence. J Clin Epidemiol. 2011;64:401–6.
Feng J, Li Z, Tian L, Mu P, Hu Y, Xiong F, et al. Efficacy and safety of curcuminoids alone in alleviating pain and dysfunction for knee osteoarthritis: a systematic review and meta-analysis of randomized controlled trials. BMC Complement Med Ther. 2022;22:276.
Luo D, Wan X, Liu J, Tong T. Optimally estimating the sample mean from the sample size, median, mid-range, and/or mid-quartile range. Stat Methods Med Res. 2018;27:1785–805.
Article MathSciNet PubMed Google Scholar
Wan X, Wang W, Liu J, Tong T. Estimating the sample mean and standard deviation from the sample size, median, range and/or interquartile range. BMC Med Res Methodol. 2014;14:135.
Nakagawa S, Noble DW, Senior AM, Lagisz M. Meta-evaluation of meta-analysis: ten appraisal questions for biologists. BMC Biol. 2017;15:18.
Anzures-Cabrera J, Higgins JP. Graphical displays for meta-analysis: an overview with suggestions for practice. Res Synth Methods. 2010;1:66–80.
Abbott JH, Robertson MC, Chapple C, Pinto D, Wright AA, De La Barra SL, et al. Manual therapy, exercise therapy, or both, in addition to usual care, for osteoarthritis of the hip or knee: a randomized controlled trial. 1: clinical effectiveness. Osteoarthritis Cartilage. 2013;21:525–34.
Chiranthanut N, Hanprasertpong N, Teekachunhatean S. Thai massage, and Thai herbal compress versus oral ibuprofen in symptomatic treatment of osteoarthritis of the knee: a randomized controlled trial. Biomed Res Int. 2014;2014: 490512.
Dong W, Zhang Q, Song M, et al. Clinical study on the treatment of early and medium stages of knee osteoarthritis withthe Xiao-Ding ointment and ‘Roujin Yangjing’ manipulative therapy. CJTCMP. 2021;36:4368–71.
Feng J. Comparison of clinical efficacy between acupuncture and manipulation in the treatment of osteoarthritis of the knee joint. Asia-Pac Tradit Med. 2014;10:80–1.
Fitzgerald GK, Fritz JM, Childs JD, Brennan GP, Talisa V, Gil AB, et al. Exercise, manual therapy, and use of booster sessions in physical therapy for knee osteoarthritis: a multi-center, factorial randomized clinical trial. Osteoarthritis Cartilage. 2016;24:1340–9.
Lai ZH, Li C, Ye C. Efficacy of spaced ginger moxibustion with tui-na therapy in the treatment of middle-aged and elderly patients with knee osteoarthritis. J Guangzhou Univ Tradit Chin Med. 2020;37:485–90.
Liang Z, Yu ZY, Yan L. Clinical randomized controlled study of six-step manipulation and electroacupuncture for early knee osteoarthritis. J Tradit Chin Med. 2012;53:1478–81.
Lin X, Wang J, Chen B, et al. Clinical study on the treatment of knee osteoarthritis by Shi’s injury Tui na rectification technique combined with red cinnamon tincture application. Chin J Tradit Chin Med. 2018;36:23–6.
Liu T, Zhang H. Clinical observation on the treatment of knee osteoarthritis by Wei’s injury technique combined with application and rubbing method. Sichuan Tradit Chin Med. 2019;37:212–4.
Peng ZW, Jiang XZ, Tang L, et al. Manual method combined with sodium glacial acid for the treatment of knee osteoarthritis. Chin J Tradit Chin Med Orthop Traumatol. 2018;26:27–30.
Perlman AI, Sabina A, Williams AL, Njike VY, Katz DL. Massage therapy for osteoarthritis of the knee: a randomized controlled trial. Arch Intern Med. 2006;166:2533–8.
Perlman AI, Ali A, Njike VY, Hom D, Davidi A, Gould-Fogerite S, et al. Massage therapy for osteoarthritis of the knee: a randomized dose-finding trial. PLoS ONE. 2012;7: e30248.
Article ADS CAS PubMed PubMed Central Google Scholar
Qu CZ, Xue P, Chen B. Effect and efficacy evaluation of tuina therapy on serum type II collagen carboxy-terminal peptide in osteoarthritis of the knee. Liaoning J Tradit Chin Med. 2020;47:170–2.
CAS Google Scholar
Wu H, Yan P, Liu WG, et al. A randomized controlled study on the treatment of knee osteoarthritis with autogenous quadriceps release manipulation. Massage Rehabil Med. 2015;6:18–9.
Xu F, Kang BX, Zhong S, et al. Combination of local acupoint pressure and seated knee adjustment method for the treatment of knee osteoarthritis: a randomized controlled study. Chin Tissue Eng Res. 2021;25:216–21.
Yao CC, Wu YH. Clinical observation of osteoarthritis of the knee joint treated with tui na based on the theory of replacing bone with tendon. New Chin Med. 2018;50:101–4.
Yuan P, Fu S. A randomized, positive drug-parallel controlled clinical study on the treatment of knee osteoarthritis based on the “holistic concept” of tuina. Shanghai J Tradit Chin Med. 2018;52:60–2+6.
Zhang D, Lv Y, Qu J, et al. A comparative study on the clinical efficacy of tui na manipulation and acupuncture in the treatment of osteoarthritis of the knee joint. Hainan Med. 2014;25:661–3.
ADS Google Scholar
Zhang L, Dong X, Wang W. Clinical efficacy of acupuncture combined with tuina in the treatment of knee osteoarthritis. Hebei Tradit Chin Med. 2018;40:763–5.
Yang S, Meng L, Zhao Y, et al. Clinical study of electroacupuncture combined with tuina in the treatment of osteoarthritis of the knee joint. China TCM Emerg. 2022;31:1177–80+92.
Xu X, Ma L, Qi X, et al. Clinical study on the treatment of wind-cold-damp paralysis type knee osteoarthritis with fire dragon pot under the theory of tendon injury. China Mod Physician. 2022;60:155–7+61.
Ma H, Wei W, Gu C, et al. Clinical efficacy of four-step and nine-phase massage in the treatment of osteoarthritis of the knee. J Tradit Chin Med. 2022;31:91–3.
Liu Y, Deng J, Sun X, et al. Effects of foot-Yangming meridian manipulation therapy on mechanical properties of quadriceps muscle and iron death of chondrocytes in patients with osteoarthritis of knee joint. Chin J Tradit Chin Med. 2022;37:5504–7.
Liu K, Zhan Y, Zhang Y, Zhao Y, Chai Y, Lv H, et al. Efficacy and safety of tuina (Chinese therapeutic massage) for knee osteoarthritis: a randomized, controlled, and crossover design clinical trial. Front Med (Lausanne). 2023;10: 997116.
Guo D, Ma S, Zhao Y, Dong J, Guo B, Li X. Self-administered acupressure and exercise for patients with osteoarthritis: a randomized controlled trial. Clin Rehabil. 2022;36:350–8.
Galbraith RF. A note on graphical presentation of estimated odds ratios from several clinical trials. Stat Med. 1988;7:889–94.
Lee RD, Tuljapurkar S. Stochastic population forecasts for the United States: beyond high, medium, and low. J Am Stat Assoc. 1994;89(1):175–89.
Sedgwick P, Marston L. How to read a funnel plot in a meta-analysis. BMJ. 2015;351: h4718.
Beaton DE. Understanding the relevance of measured change through studies of responsiveness. Spine (Phila Pa 1976). 2000;25:3192–9.
Tubach F, Ravaud P, Martin-Mola E, Awada H, Bellamy N, Bombardier C, et al. Minimum clinically important improvement and patient acceptable symptom state in pain and function in rheumatoid arthritis, ankylosing spondylitis, chronic back pain, hand osteoarthritis, and hip and knee osteoarthritis: results from a prospective multinational study. Arthritis Care Res (Hoboken). 2012;64:1699–707.
Dai WL, Lin ZM, Guo DH, Shi ZJ, Wang J. Efficacy and safety of hylan versus hyaluronic acid in the treatment of knee osteoarthritis. J Knee Surg. 2019;32:259–68.
Wu Q, Zhao J, Guo W. Efficacy of massage therapy in improving outcomes in knee osteoarthritis: a systematic review and meta-analysis. Complement Ther Clin Pract. 2022;46: 101522.
Xu X, Liu W, Xu S, Li Y, Zhang QW, Huang HX. Clinical practice guidelines for knee osteoarthritis in integrated traditional Chinese and Western medicine. J Pract Med. 2021;37:2827–33.
Da Costa BR, Saadat P, Basciani R, Agarwal A, Johnston BC, Jüni P. Visual analogue scale has higher assay sensitivity than WOMAC pain in detecting between-group differences in treatment effects: a meta-epidemiological study. Osteoarthritis Cartilage. 2021;29:304–12.
Bjerre-Bastos JJ, Miller CP, Li Y, Andersen JR, Karsdal M, Bihlet AR. Associations between single-question visual analogue scale pain score and weight-bearing and non-weight-bearing domains of Western Ontario and McMaster Universities Arthritis Index pain: data from 2 phase 3 clinical trials. Pain Rep. 2022;7:1017.
Rubinstein SM, De Zoete A, Van Middelkoop M, Assendelft WJJ, De Boer MR, Van Tulder MW. Benefits and harms of spinal manipulative therapy for the treatment of chronic low back pain: systematic review and meta-analysis of randomised controlled trials. BMJ. 2019;364: l689.
Weerapong P, Hume PA, Kolt GS. The mechanisms of massage and effects on performance, muscle recovery and injury prevention. Sports Med. 2005;35:235–56.
Zhang H, Zhao M, Wu Z, Wang X, Jiang Y, Liang J, et al. Effects of acupuncture, moxibustion, cupping, and massage on sports injuries: a narrative review. Evid Based Complement Alternat Med. 2022;2022:9467002.
PubMed PubMed Central Google Scholar
Download references
Acknowledgements
We would like to thank Guangxin Guo from the Shanghai University of Traditional Chinese Medicine for their contributions and constructive comments in developing the prephase literature search and study selection used in this review.
This study was supported by the National Natural Science Foundation of China (No. 81973973), the Clinical Research Plan of SHDC (SHDC2020CR4055), the Support Program for Chinese Medicine Innovation Teams and Talents of the State Administration of Traditional Chinese Medicine (ZYYCXTD-C-202008), the Construction of Research-oriented Wards of SHDC (SHDC2022CRW010), and the Three-Year Action Plan to Promote Clinical Skills and Innovation Capabilities of SHDC (SHDC2022CRT018).
Author information
Bowen Zhu and He Ba contributed equally to this work.
Authors and Affiliations
Shuguang Hospital, Shanghai University of Traditional Chinese Medicine, Shanghai, China
Bowen Zhu, Lingjun Kong, Jun Ren & Min Fang
Department of Integrative Oncology, Shanghai Cancer Center, Qingdao Institute, Fudan University, Qingdao, China
Department of Traditional Chinese Massage, Yueyang Hospital of Integrated Traditional Chinese and Western Medicine, Shanghai University of Traditional Chinese Medicine, Shanghai, China
Yangyang Fu & Qingguang Zhu
Institute of Traditional Chinese Medicine and Massage, Shanghai Academy of Traditional Chinese Medicine, Shanghai, China
Qingguang Zhu & Min Fang
You can also search for this author in PubMed Google Scholar
Contributions
The conception and design of this study were performed by MF and BZ. HB and BZ collected the data. Risks-of-bias assessments were conducted by LK and RJ. QZ and LK screened all potentially eligible studies. Data acquisition, analysis, and interpretation were performed by HB and BZ. The manuscript was drafted by HB and BZ. MF supervised this study and critically revised the manuscript for intellectual content. QZ and YF were responsible for the integrity of the entire work.
Corresponding authors
Correspondence to Qingguang Zhu or Min Fang .
Ethics declarations
Ethics approval and consent to participate.
Not applicable.
Consent for publication
Competing interests.
The authors declare that they have no competing interests.
Additional information
Publisher’s note.
Springer Nature remains neutral with regard to jurisdictional claims in published maps and institutional affiliations.
Supplementary Information
Additional file 1:.
Appendix 1. Search terms and strategies. Appendix 2. Detailed risk of bias judgment by domains for Stress (Rob2 Tool): at the end of the intervention. Appendix 3. The GRADE approach to evidence synthesis and operationalization of criteria items. Appendix 4. Funnel plot for VAS score. Appendix 5. Sensitivity analysis for VAS score. Appendix 6. Meta-regression (Table S1–5).
Rights and permissions
Open Access This article is licensed under a Creative Commons Attribution 4.0 International License, which permits use, sharing, adaptation, distribution and reproduction in any medium or format, as long as you give appropriate credit to the original author(s) and the source, provide a link to the Creative Commons licence, and indicate if changes were made. The images or other third party material in this article are included in the article's Creative Commons licence, unless indicated otherwise in a credit line to the material. If material is not included in the article's Creative Commons licence and your intended use is not permitted by statutory regulation or exceeds the permitted use, you will need to obtain permission directly from the copyright holder. To view a copy of this licence, visit http://creativecommons.org/licenses/by/4.0/ . The Creative Commons Public Domain Dedication waiver ( http://creativecommons.org/publicdomain/zero/1.0/ ) applies to the data made available in this article, unless otherwise stated in a credit line to the data.
Reprints and permissions
About this article
Cite this article.
Zhu, B., Ba, H., Kong, L. et al. The effects of manual therapy in pain and safety of patients with knee osteoarthritis: a systematic review and meta-analysis. Syst Rev 13 , 91 (2024). https://doi.org/10.1186/s13643-024-02467-7
Download citation
Received : 10 June 2023
Accepted : 21 January 2024
Published : 19 March 2024
DOI : https://doi.org/10.1186/s13643-024-02467-7
Share this article
Anyone you share the following link with will be able to read this content:
Sorry, a shareable link is not currently available for this article.
Provided by the Springer Nature SharedIt content-sharing initiative
- Manual therapy
- Knee osteoarthritis
- Meta-analysis
Systematic Reviews
ISSN: 2046-4053
- Submission enquiries: Access here and click Contact Us
- General enquiries: [email protected]
Osteoarthritis: Pathology, Diagnosis, and Treatment Options
Affiliations.
- 1 Department of PM&R, University of Pennsylvania, Penn Medicine Rittenhouse, 1800 Lombard Street, Philadelphia, PA 19146, USA.
- 2 Department of PM&R, University of Pennsylvania, Penn Medicine Rittenhouse, 1800 Lombard Street, Philadelphia, PA 19146, USA. Electronic address: [email protected].
- PMID: 32035570
- DOI: 10.1016/j.mcna.2019.10.007
Osteoarthritis (OA) is a worldwide endemic and debilitating disease. Previously thought to simply be damaged from "wear and tear," OA is now understood to be a complex interaction of local and systemic factors. This article reviews the pathology, symptoms, diagnosis, and various conservative, surgical, and novel treatments of OA.
Keywords: Arthralgia; Arthritis; Degenerative joint disease; Osteoarthritis.
Copyright © 2019 Elsevier Inc. All rights reserved.
Publication types
- Osteoarthritis* / diagnosis
- Osteoarthritis* / physiopathology
- Osteoarthritis* / therapy
- Patient Care Management / methods*
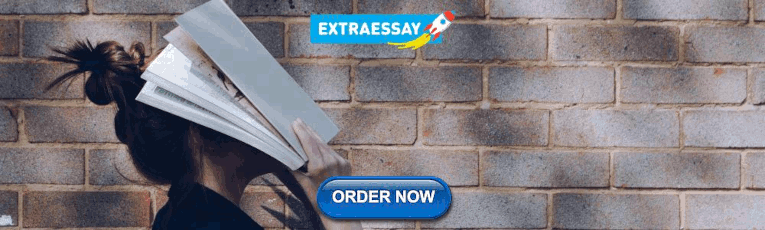
IMAGES
VIDEO
COMMENTS
Abstract. Osteoarthritis (OA) has a very high incidence worldwide and has become a very common joint disease in the elderly. Currently, the treatment methods for OA include surgery, drug therapy, and exercise therapy. In recent years, the treatment of certain diseases by exercise has received increasing research and attention.
The mechanism that exercise alleviates the pathological changes of OA by affecting the degradation of the ECM, apoptosis, inflammatory response, autophagy, and changes of ncRNA is expounded, and the effects of different exercise types on OA patients are summarized. Osteoarthritis (OA) has a very high incidence worldwide and has become a very common joint disease in the elderly. Currently, the ...
Exercise for Osteoarthritis: A Literature Review of Pathology and Mechanism Hui Kong, Xue-Qiang Wang, Xue-Qiang Wang, Xin-An Zhang; Affiliations Hui Kong College of Kinesiology, Shenyang Sport University, Shenyang, China Xue-Qiang Wang Department of Sport Rehabilitation, Shanghai University of Sport, Shanghai, China ...
In this review, we reviewed the possible pathological mechanisms of OP and summarized the effects of exercise on bone metabolism, and the mechanisms by which exercise alleviates the progression of OP, to provide a reference for the prevention and treatment of OP. Keywords: osteoporosis, exercise, inflammatory reaction, cytokines, mechanical stress.
The aim of this literature review is to review the existing evidence regarding the impact of exercise in people with knee osteoarthritis concerning physical and functional outcomes. The secondary aim is to provide both healthcare professionals and patients with updated and high-quality recommendations for the management of this condition.
Background: Exercise is an effective nonpharmaceutical therapy for knee osteoarthritis (KOA). Purpose: To identify the most effective type of exercise therapy for KOA with regard to pain, stiffness, joint function, and quality of life. Study design: Systematic review; Level of evidence, 3. Methods: The PubMed, Web of Science, Embase, and Cochrane Library databases were searched, from inception ...
Bone Research (2023) Osteoarthritis (OA) is the most common degenerative joint disease that causes painful swelling and permanent damage to the joints in the body. The molecular mechanisms of OA ...
Impact of exercise type and dose on pain and disability in knee osteoarthritis: A systematic review and meta-regression analysis of randomized controlled trials: Article: 10.995: 8: 103: 0.01: Fransen M (2015) Br J Sport Med: Exercise for osteoarthritis of the knee: A Cochrane systematic review: Review: 13.8: 9: 77: 0.03: Zhang W (2010 ...
Osteoarthritis (OA) is the most common degenerative joint disease and a major cause of pain and disability in adult individuals. The etiology of OA includes joint injury, obesity, aging, and heredity. However, the detailed molecular mechanisms of OA initiation and progression remain poorly understood and, currently, there are no interventions ...
The possible mechanisms are that exercise affects apoptosis and autophagy through the release of exercise-stimulated myohormone and the secretion of anti-inflammatory cytokines via mechanical force. In addition, exercise may also have an impact on the epigenetic processes involved in bone metabolism. Mechanical stimulation promotes bone marrow ...
and exercise frequency have different effects on OA patients. At the same time, suitable exercise prescriptions are recommended for OA patients. Keywords: osteoarthritis, exercise, pathology, mechanism, therapy INTRODUCTION Osteoarthritis (OA) has a very high incidence worldwide, and it is strongly associated with age (van Saase et al.,1989).
Abstract and Figures. Osteoarthritis (OA) has a very high incidence worldwide and has become a very common joint disease in the elderly. Currently, the treatment methods for OA include surgery ...
Abstract. Osteoarthritis (OA), the most prevalent chronic joint disease, increases in prevalence with age, and affects majority of individuals over the age of 65 and is a leading musculoskeletal cause of impaired mobility in the elderly. Because the precise molecular mechanisms which are involved in the degradation of cartilage matrix and ...
Figure 2 The mechanism of exercise improving osteoporosis. Exercise-induced changes in mechanical stress, hormones, and cytokines can regulate the pathological changes of osteoporosis. Mechanistically, exercise reduces the harmful osteoporosis alterations via affecting apoptosis, inflammatory response, and autophagy, and exercise may affect the epigenetic mechanisms of bone metabolism by ...
(B) Mechanism of pathological changes of OA through ncRNA. from publication: Exercise for Osteoarthritis: A Literature Review of Pathology and Mechanism | Osteoarthritis (OA) has a very high ...
Osteoarthritis (OA) has a very high incidence worldwide and has become a very common joint disease in the elderly. Currently, the treatment methods for OA include surgery, drug therapy, and exercise therapy. In recent years, the treatment of certain diseases by exercise has received increasing research and attention. Proper exercise can improve the physiological function of various organs of ...
The possible pathological mechanisms of OP are reviewed and summarized the effects of exercise on bone metabolism, and the mechanisms by which exercise alleviates the progression of OP, to provide a reference for the prevention and treatment of OP. Osteoporosis (OP) is a disease that weakens bones and has a high morbidity rate worldwide, which is prevalent among the elderly, particularly ...
Osteoarthritis and exercise: a review of the literature. Osteoarthritis and exercise: a review of the literature. Osteoarthritis and exercise: a review of the literature J S C Med Assoc. 2009 Feb;105(1):8-11. Authors Emily Keeling Farrar 1 , Holly Mitchell. Affiliation 1 ...
1. Introduction. Osteoarthritis (OA) is the most common progressive musculoskeletal condition that can affect joints, but it mainly affects the hips and knees as predominant weight-bearing joints [1,2,3].Knee osteoarthritis is characterized by structural modifications to primarily articular cartilage and the subchondral bone, but also Hoffa's fat pad, synovia, ligaments and muscles, leading ...
Pathological Change Mechanism of Osteoarthritis. For OA patients, the integrity of the entire joint tissue is damaged, including articular cartilage, subchondral bone, and synovial membrane (Loeser et al., 2012).Articular cartilage is mainly composed of the extracellular matrix (ECM), consisting of water, collagen, proteoglycans, mucopolysaccharides, type II collagen, and chondrocytes (Luo et ...
Knee osteoarthritis (KOA) is a chronic degenerative disease of the knee joint, and knee joint pain is the most common clinical manifestation [].The worldwide prevalence of radiographically confirmed symptomatic KOA is estimated to be 3.8%, and the prevalence of this disease has increased to more than 10% in people older than 60 years [].In China, the prevalence of KOA among older people is ...
Abstract. Osteoarthritis (OA) is a worldwide endemic and debilitating disease. Previously thought to simply be damaged from "wear and tear," OA is now understood to be a complex interaction of local and systemic factors. This article reviews the pathology, symptoms, diagnosis, and various conservative, surgical, and novel treatments of OA.
Abstract. Patients with osteoarthritis (OA) are faced with a barrage of treatment options, from recommendations from friends and social media to medications prescribed by the primary care physician. The purpose of this article is to critically review current approaches to generalized or monoarticular OA based on available evidence and to ...