- Important Notices
- GBI Secure Login

- Program and Exams
- Fees and Payments
- Our FRM Certified Professionals
- Study Materials
- Exam Logistics
- Exam Policies
- Risk Career Blog
- Register for FRM Exam
- Path to Certificate
- Climate Resource Center
- Foundations of Financial Risk (FFR)
- Financial Risk and Regulation (FRR)
- About Membership
- Exclusive Offers
- Risk Intelligence
- Board of Trustees
- GARP Benchmarking Initiative (GBI)
- GARP Risk Institute (GRI)
- Buy Side Risk Managers Forum
- Academic Partners
- Careers at GARP
- Culture & Governance
- Sustainability & Climate
- Operational
- Comment Letters
- White Papers
- Islamic Finance Book
Physical Risk - Risk Management - Transition Risk
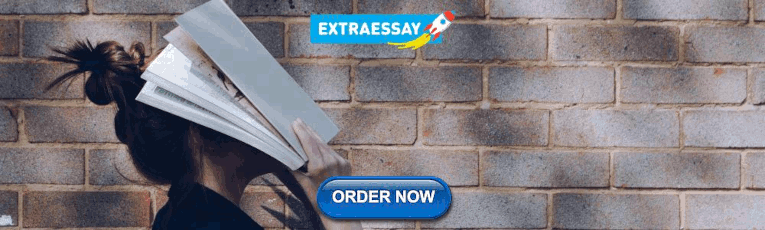
India: A Case Study in Climate Mitigation and Adaptation
This article explores the difficult trade-offs that need to be made between the competing claims of climate mitigation, adaptation, and economic development..
Thursday, September 14, 2023
By Maxine Nelson
This article has been extensively updated, incorporating new COP 27 commitments, Reserve Bank of India (RBI) statements and current green bond issuance. It was originally published Oct. 18, 2021.
After decades of population growth and economic development, India is now the third largest emitter of greenhouse gases in the world. In addition, India is among the countries most vulnerable to climate change due to its geography and dependence on agriculture.
It has been estimated that if emissions are not significantly reduced, India could suffer economic losses of USD 35 trillion . Indeed, much of India has been experiencing annual heatwaves followed by intense flooding, and in 2021 alone it experienced even more extreme weather events — including cyclones and a glacier collapse. Thus, India makes a thought-provoking case study for policymakers and risk professionals given the difficult trade-offs that need to be made between the competing claims of climate mitigation, adaptation and economic development.
Climate Change’s Effect on India
The banking regulator, Reserve Bank of India (RBI) , explains that “India has witnessed changes in climatic patterns in line with the rest of the world… the rainfall pattern, particularly with respect to the [south west monsoon] SWM, which provides around 75 percent of the annual rainfall, has undergone significant changes. Moreover, the occurrence of extreme weather events like floods/unseasonal rainfall, heat waves and cyclones has increased during the past two decades, and data reveal that some of the key agricultural states in India have been the most affected by such events.”
A more recent, detailed RBI study points out that “it is the increased frequency of extreme weather occurrences that is breaking the back of our capability to cope with natural disasters.” As shown by India’s nationally determined contributions (NDCs) — the actions it has committed to take to reduce its emissions and adapt to the impacts of climate change — it is among the most vulnerable countries in the world to the impact of accelerated sea level rise from global warming. This is due to its long coastline, large number of islands and population of 170 million living in coastal regions.
The RBI also notes that precipitation and temperature — the two key climate indicators — “play a crucial role in the overall health of the Indian economy.” As well as affecting food production, the extreme weather in agricultural states impacts employment and GDP, with approximately 44% of the working population employed in agriculture and allied sectors which contribute about 20% of GDP, according to M.K. Jain, the deputy governor of the Reserve Bank . Several challenges confronting Indian agriculture, including diminishing and degrading natural resources and unprecedented climate change, need to be tackled for the long-term sustainability and viability of Indian agriculture.
However, there is uncertainty over how large the impacts might be. The Swiss Re Institute , for example, estimates a 35% reduction in the level of India’s GDP by 2050 if greenhouse gas emissions are not reduced globally, and approximately a 6% GDP reduction even if the Paris Agreement goals are met. An Oxford Economics report “Estimating the Economic Impact of Global Warming” has framed the impact differently, estimating that India’s GDP could be 90% lower in 2100 than it would be if there was no climate change, suggesting that climate change has the potential to absorb all of India’s future prospective growth in income per capita. And Deloitte has estimated USD 35 trillion of economic losses by 2070. While these different approaches produce diverse estimates, they all show that the impact will be big and require additional investments in both mitigation and adaptation.
India’s Effect on Climate Change
Not only will the changing climate have a significant impact on India, but India is also expected to have a significant impact on the climate. Although historically it has not had high emissions, India rose to the number three spot in the national emissions rankings 15 years ago, behind China and the U.S. The RBI noted that “With the increase in population, the cumulative level of greenhouse gas (GHG) emissions has increased, resulting in a rise of average temperature. According to a study by the International Energy Agency (IEA), India emitted 2,299 million tonnes of carbon dioxide (CO 2 ) in 2018, a rise of 4.8% over the previous year.”
Unfortunately, India’s future potential emissions are not yet aligned with the Paris Agreement goals. India’s NDCs currently correspond to temperature increases above 3°C, according to Climate Action Tracker . (You can find out more about NDCs and their place in the Paris Agreement in this short article . ) India increased its commitment to reduce greenhouse gas emissions at COP 26, the 2021 annual meeting of the signatories of the Paris Agreement, where it pledged to cut its emissions to net zero by 2070. While this was a large increase in commitment, it isn’t yet aligned with the worldwide goal of cutting emissions to net zero by 2050 needed to limit global warming to 1.5°C.
Maxine Nelson
In advance of COP 27, India has again increased its commitment and pledged to a 45% reduction in GDP emissions intensity by 2030 — marking an 10% increase from the previous pledge. Any emissions reduction is helpful to mitigate climate change. However, as the pledge is based on emissions intensity and not absolute emissions, emissions can continue increasing as the economy expands. This pledge, therefore, doesn’t meet the net-zero goal of reducing emissions by 45% by 2030. Still, the effort required to overcome the challenge of rapidly expanding an economy while decreasing emissions intensity needs to be appreciated.
To further mitigate climate change, India may need to agree to reduce its emissions even more — a big task for a developing economy with average annual energy consumption of a third the global average, and per capita emissions already 10 times lower than that of the U.S., four times lower than China, and three times lower than Europe. With IPCC reports highlighting the urgency of tackling climate change quickly to reduce the loss and damage for humans and ecosystems, it is even more important that emissions reductions are ambitious.
Financing Mitigation and Adaptation
A 2021 RBI Financial Stability Report noted that climate change and the associated mitigating policy commitments are “set to reshape the macroeconomic and financial landscape”. Extensive funding is needed both to reduce future emissions and to finance the adaptation needed to manage the impacts of climate change. In their 2016 NDC, India estimated that at least USD 2.5 trillion (at 2014-15 prices) would be required for meeting its climate change actions between 2016 and 2030. And the International Energy Agency estimates that nearly 60% of India’s CO 2 emissions in the late 2030s will be coming from infrastructure and machines that do not exist today. If this investment is to be sustainable, USD 1.4 trillion extra funding (above that required for current policies) is needed over the next 20 years.
Like most of the world, green bond issuance in India — which could provide some of this funding — is currently a small proportion of all bond issuance. The rate of issuance is increasing, however, with USD 21.6 billion of green, sustainable or social bonds issued in 2022. And in 2023, the Government of India entered the green finance market issuing USD 2 billion of green bonds to finance their spending on a range of projects including solar power, green hydrogen and afforestation. As they obtained a greenium (lower financing costs than other equivalent bonds), we should expect to see more of these issued in the future.
There are also substantial opportunities in other financial markets, such as the development of a derivatives market to aid adaptation via products such as:
- agricultural commodity derivatives, which can help reduce risks by enabling continuous price discovery and providing hedging
- weather derivatives, which can hedge the risks of high-probability, low-risk events
Of course, meeting the needs of climate change financing carries the usual financial risk implications of any lending. An RBI analysis shows that banks’ direct exposure to fossil fuels (through electricity, chemicals and cars) is 10% of total outstanding non-retail bank credit, so it should have a limited impact on the banking system. However, it notes that many other industries indirectly use fossil fuels and their impacts also need to be closely monitored.
Regulatory Response
The RBI has noted that policy measures such as a deepening of the corporate bond market, standardization of green investment terminology, consistent corporate reporting and removing information asymmetry between investors and recipients can make a significant contribution in addressing some of the shortcomings of the green finance market.
Like in most of the rest of the world, there is an increasing regulatory focus on climate risk. The RBI Governor has stated that guidelines will be issued about disclosure of climate-related risks, and also scenario analysis and stress testing. This followed last year’s RBI consultation which asked for inputs on a comprehensive range of topics from climate risk governance to strategy, and risk monitoring, management and mitigation at regulated entities. This consultation, in turn, built on the results of an RBI survey of banks that was also published last year. The survey found that “although banks have begun taking steps in the area of climate risk and sustainable finance, there remains a need for concerted effort and further action in this regard.” It also found that board-level engagement is inadequate, and few banks had a strategy for incorporating climate risk into their risk management framework. To see what leading climate risk firms are doing globally look at GARP’s whitepaper: “ Climate Risk Leadership: Lessons From 4 Annual Surveys .”
Given the widespread impact of climate change, it isn’t just the banking regulator that is looking at how climate risk will affect firms in its jurisdiction. In 2021, the Securities and Exchange Board of India (SEBI) mandated that the largest 1,000 listed firms complete a Business Responsibility and Sustainability Report . The report asks for information like material ESG risks and opportunities and their financial implications; sustainability related targets and performance; and their greenhouse gas emissions. Companies’ value chains also need to be assessed. This requirement is being progressively rolled out from 2023 to 2027, with the largest companies also required to get assurance of their disclosures.
In addition, SEBI has altered the rules for mutual funds , allowing them to have multiple ESG schemes with different strategies; in the past, a mutual fund could only have one ESG fund. This increase in scope follows one for green debt securities , which was expanded to include bonds such as blue bonds (sustainable water management and marine sector), yellow bonds (solar energy generation and transmission), transition bonds and adaptation bonds. Both of these expansions in scope should increase financing for sustainability related initiatives.
Reflecting the fact that addressing climate change is a global problem, needing both local and global solutions, the RBI joined the Network for Greening the Financial System (NGFS) in April 2021. The NGFS’s purpose is to strengthen the global response required to meet the goals of the Paris Agreement and to enhance the role of the financial system to manage risks and to mobilize capital for green and low-carbon investments. These goals align very well with the work India needs to undertake to make not just its financial system resilient to the risks from climate change, but to balance mitigation, adaptation, and economic development across the country.
Maxine Nelson , Ph.D, Senior Vice President, GARP Risk Institute, currently focusses on sustainability and climate risk management. She has extensive experience in risk, capital and regulation gained from a wide variety of roles across firms including Head of Wholesale Credit Analytics at HSBC. She also worked at the U.K. Financial Services Authority, where she was responsible for counterparty credit risk during the last financial crisis.
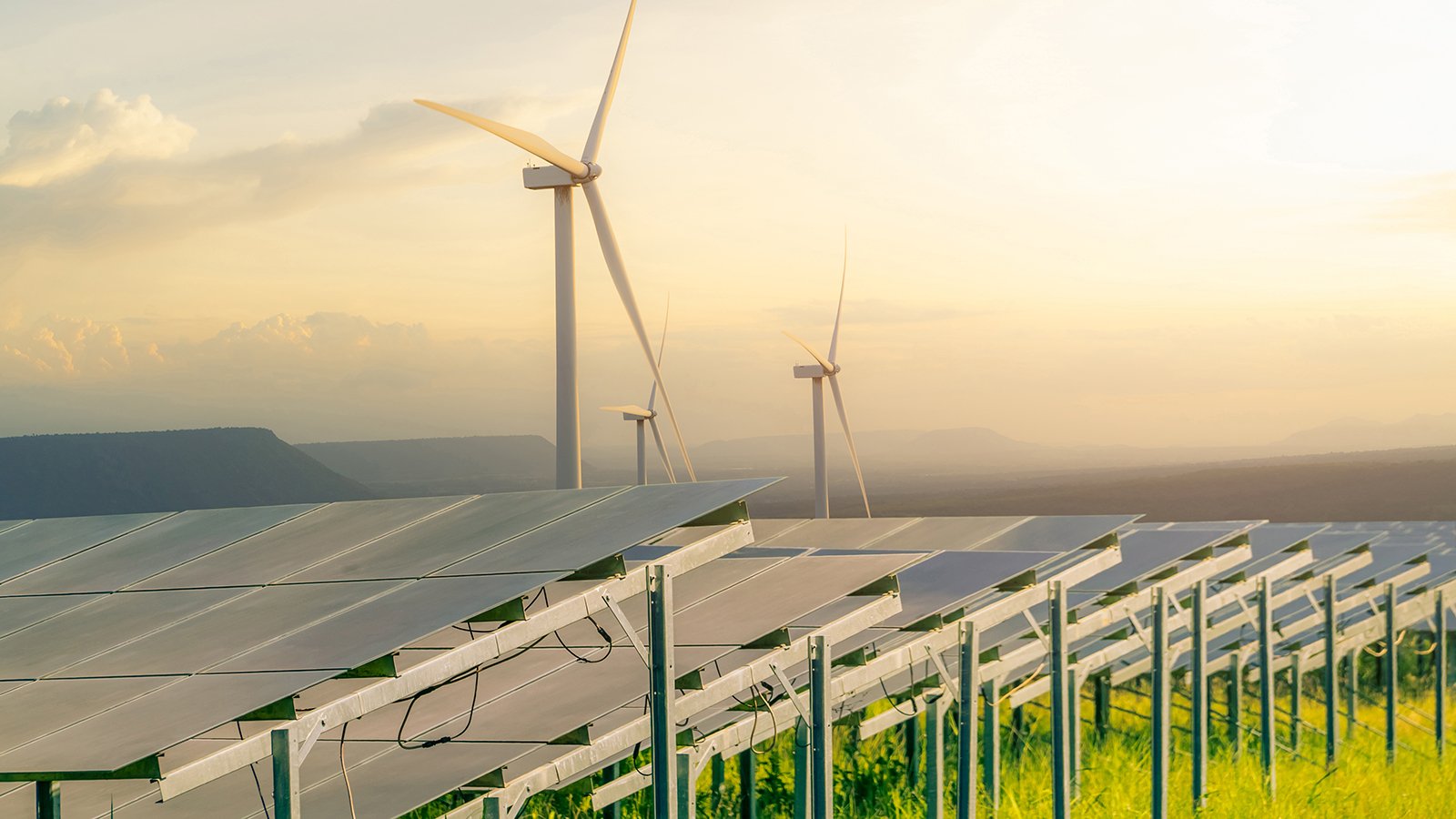
ESG Explained: What It Is and Why It Matters Jun 30, 2023
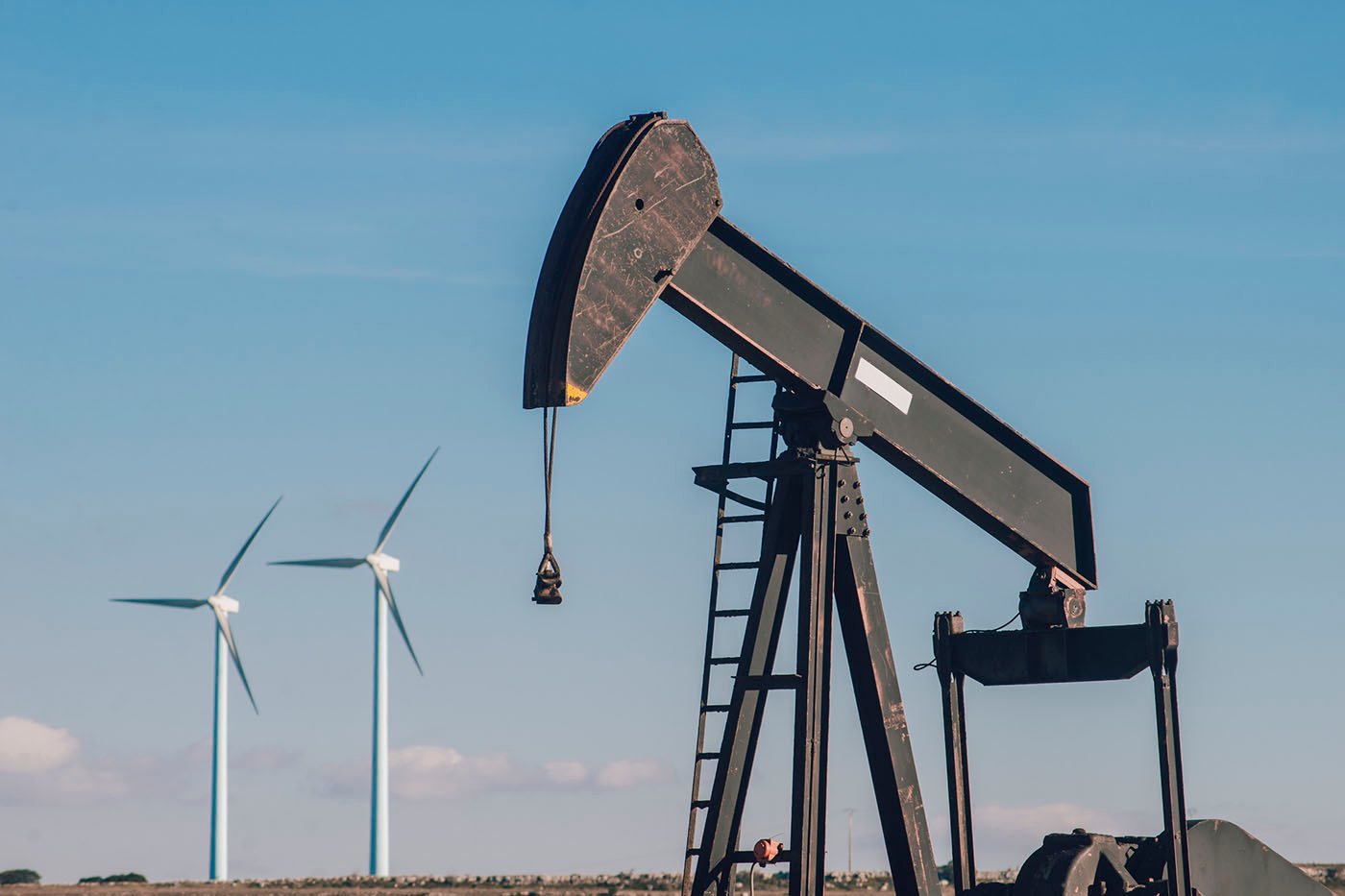
The 4 Main Drivers of Transition Risk, and Why the Risks Are Increasing Apr 12, 2022
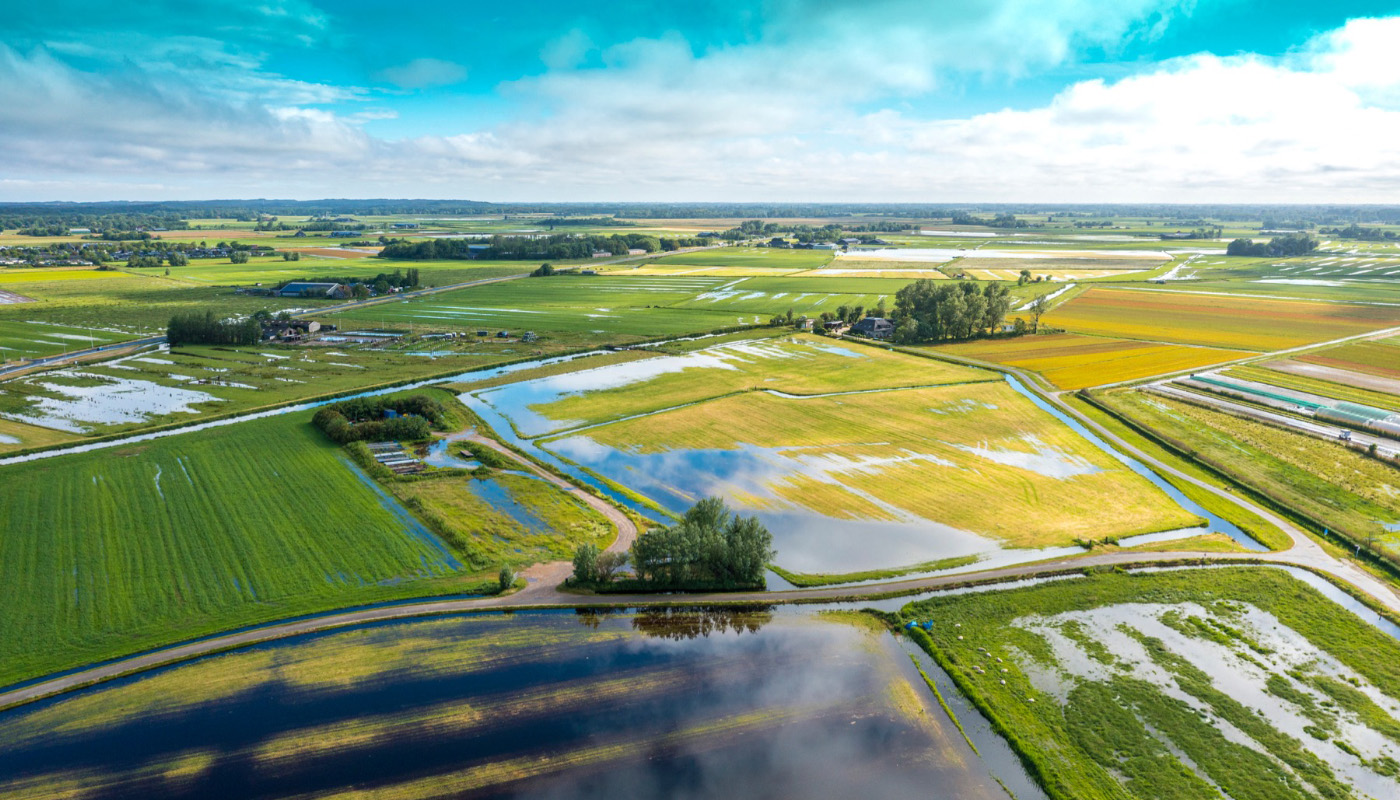
Understanding the Physical Risks Associated with Climate Change Apr 27, 2022
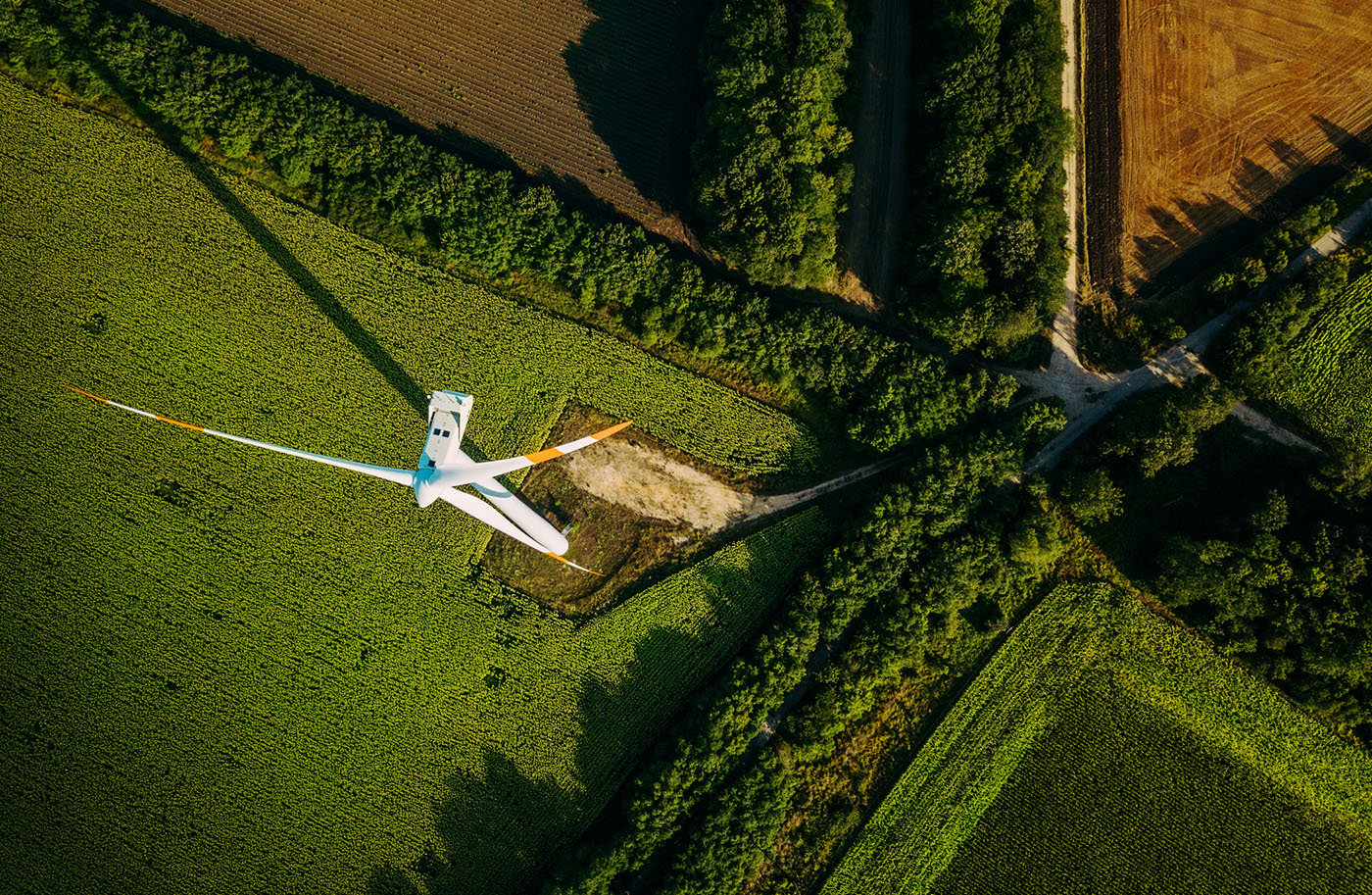
4 Key Areas to Know About Climate Change May 18, 2023
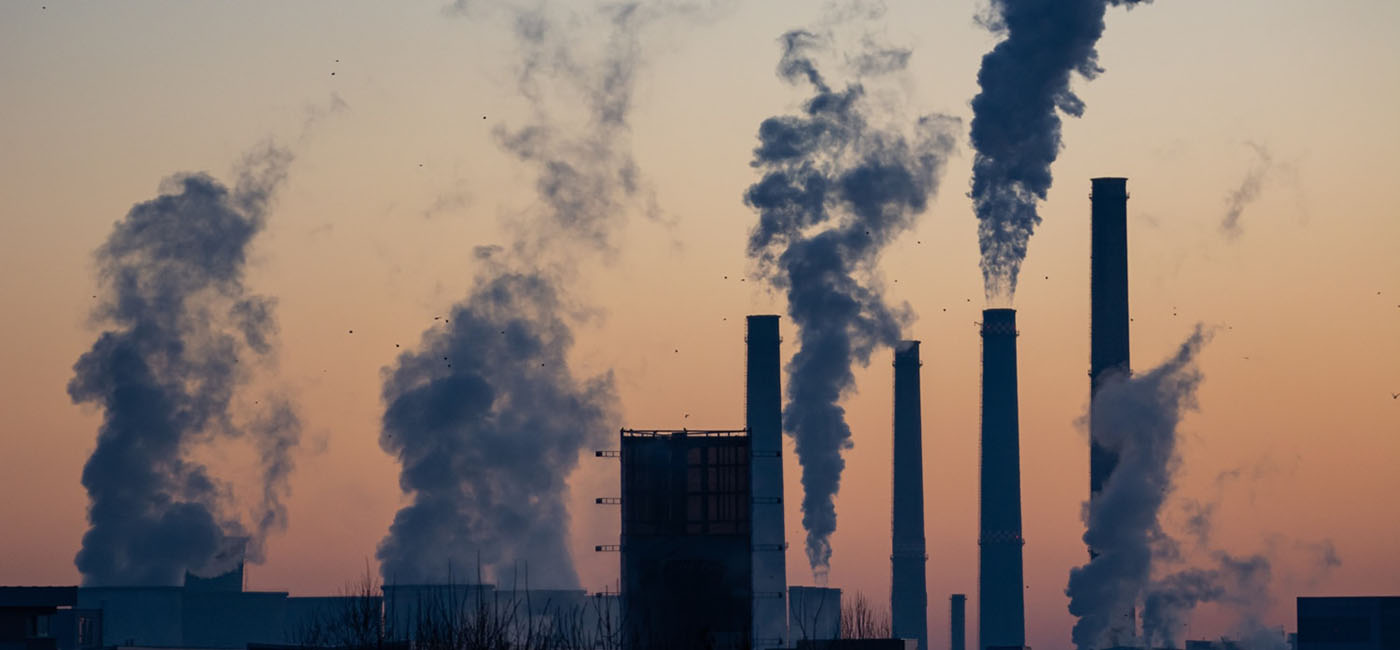
India: A Case Study in Climate Mitigation and Adaptation Sep 14, 2023
- Financial Risk Manager
- Sustainability and Climate Risk
We are a not-for-profit organization and the leading globally recognized membership association for risk managers.
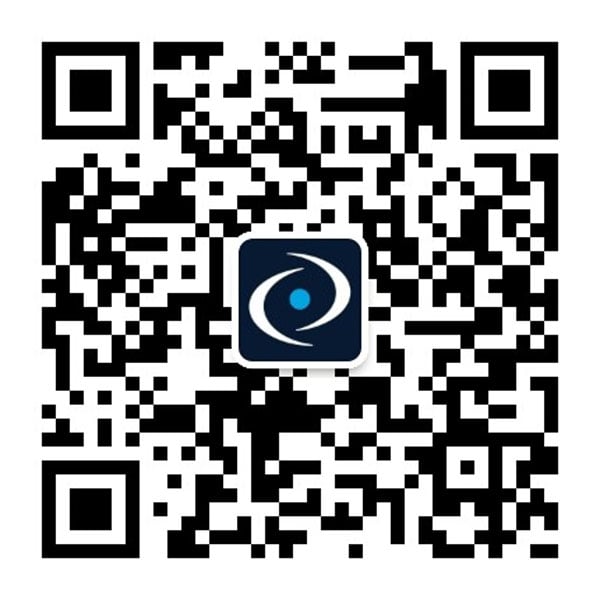
• Bylaws • Code of Conduct • Privacy Notice • Terms of Use © 2024 Global Association of Risk Professionals

- JOIN A TRAINING
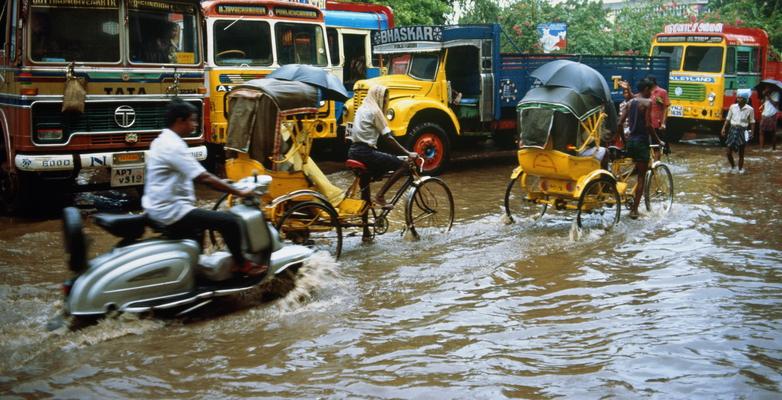
How the Climate Crisis is Impacting India
As global temperatures rise, we will continue to see india's poorest suffer the most as climate change destroys livelihoods and washes away communities. .
As India’s prime minister, Narendra Modi, said last year during remarks at the UN’s COP 26 climate conference in Glasgow, Scotland: “For many developing countries, climate change is looming large over their existence. We have to take big steps today to save the world. This is the need of the hour.”
From heat waves devastating crop yields to torrential rains causing flooding that submerges entire communities, India is experiencing some of the most extreme impacts of the climate crisis. While India comprises a little over 17% of the world’s population, it produces just about 7% of global emissions.
Here are a few major ways climate change is affecting India.
INDIA IS HEATING UP
There’s no denying it: India is heating up. Between 1901 and 2018, temperatures rose by 1.3 degrees Fahrenheit (0.7 degrees Celsius) .
This might seem miniscule, but slight increases in temperature can throw our planet’s natural systems out of whack , contributing to extreme weather like heat waves, drought, and flooding. These climate impacts also disproportionately affect working families and people of color.
In India (and all over the world), you can see the effects of rising temperatures everywhere you look as the climate crisis disrupts our daily lives – and critical sectors like our energy, agriculture, and transportation systems. This spring, India sweltered through its hottest March on record .
A heat wave lasting for weeks caused temperatures to soar above 110 degrees, with some areas hitting 115 degrees. Researchers found that between 2000-2004 and 2017-2021, India saw a 55% increase in deaths due to extreme heat . March’s heat wave killed at least 90 people across India and Pakistan, contributed to forest fires, and devastated farms and India’s wheat yield.
Before its agriculture industry was impacted, India was going to step in and help provide food relief to countries in need after the Russia-Ukraine crisis disrupted the global food system. But after the heat wave, India had to ban wheat exports to ensure its own people had enough to eat. One study found that climate change made the heat wave 30 times more likely .
FLOODING, DROUGHT, AND RISING TEMPERATURES
Like many countries around the world, India is facing water security issues, and at the same time, widespread flooding.
India’s agricultural sector relies on monsoon season. However, over the last century, there have been more days with extremely heavy rains with longer dry spells in between. This has heavily impacted India’s central belt running from western Maharashtra State to the Bay of Bengal, which has seen extreme rainfall events increase threefold over the last 70 years, but has also had a decrease in total annual rainfall .
The Himalayas, a long-time protector against drought in India, are at risk, too. A 2019 report predicted that by 2100, at least one-third of the glaciers in the region will be gone . Glacial melting as a result of rising temperatures has also exacerbated both flooding and drought, especially in agricultural mountain communities that rely on seasonal snowmelt .
Flooding impacts India’s urban areas, too. This fall, torrential rain flooded Bengaluru, known as India’s Silicon Valley, where it disrupted water supplies and left many stranded in their homes. In some areas of the city, rapid urbanization and a lack of sustainable infrastructure is thought to have contributed to the flooding.
In 2005, 37 inches of rain hit Maharasthra state in under 24 hours, flooding the city of Mumbai and killing almost 900 people. The heavy rain was traced back to warming in the Arabian Sea, which is thought to have contributed to the moisture surge. In the past decade, flooding attributed to extreme rain events resulted in losses of approximately $3 billion each year.
HOW YOU CAN FIGHT THE CLIMATE CRISIS
As global temperatures rise, we will continue to see the country’s poorest suffer the most as climate change destroys livelihoods and washes away communities. Last year, Cyclone Yaas left an estimated 150,000 homeless in eastern India after destroying at least 25,000 homes.
In India, approximately 70% of households rely on agriculture for their livelihoods but are “struggling with lower yields” attributed to “frequent droughts and lower rainfall.” Farmers are severely impacted by these unpredictable weather patterns, which has caused many to move on to urban areas, where extreme heat can make outdoor work dangerous. These are just a few ways the climate crisis hits India’s most vulnerable communities the hardest.
The good news is that there’s still time to turn things around. But only if we act now. Here at Climate Reality, we are fighting to bring real solutions to communities all over the world, and with support from activists like you, we’re catalyzing the bold climate action that our planet desperately needs.
You can get even more involved in our work to forge a just, sustainable future by joining one of our upcoming trainings and becoming a Climate Reality Leader. You’ll join a global community with the skills and knowledge to take on the climate crisis – and win.
To stay connected with the fight for climate solutions around the world, sign up for our email activist list today .
MORE FROM OUR BLOG
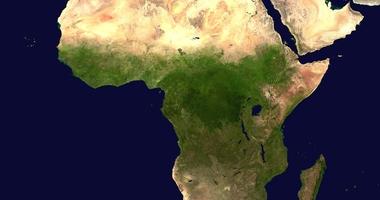
Africa: Not Your Gas Station
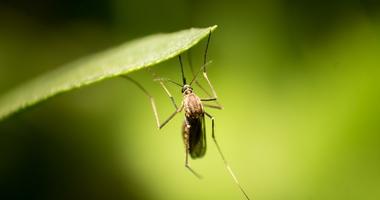
New Diseases Popping Up In Your Area? Blame Climate Change and Deforestation
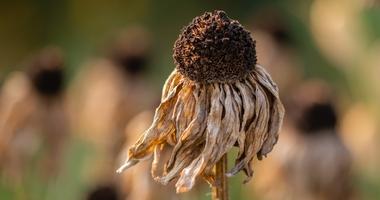
Just Say It: The Climate Crisis is Already Killing Us
- Reference Manager
- Simple TEXT file
People also looked at
Review article, navigating the impact of climate change in india: a perspective on climate action (sdg13) and sustainable cities and communities (sdg11).
- 1 Department of Environmental Science, Miranda House College, University of Delhi, New Delhi, India
- 2 Department of Botany, Aligarh Muslim University, Aligarh, India
- 3 Department of Environmental Science, Hindu College, University of Delhi, New Delhi, India
- 4 Air Quality Sustainable Cities & Transport, WRI India Ross Center for Sustainable Cities and Transport, Mumbai, India
- 5 Department of Environmental Studies, Zakir Husain Delhi College, University of Delhi, New Delhi, India
Climate change is a global concern of the current century. Its rapid escalation and ever-increasing intensity have been felt worldwide, leading to dramatic impacts globally. The aftermath of climate change in India has brought about a profound transformation in India's environmental, socio-economic, and urban landscapes. In 2019, India ranked seventh, among the most affected countries by extreme weather events caused due to changing climate. This impact was evident in terms of both, the human toll with 2,267 lives lost, and the economic damage, which accounted for 66,182 million US$ Purchasing power parities (PPPs). Over the recent years, India has experienced a significant increase in the number and frequency of extreme weather events, causing vulnerable communities. The country experienced severe air pollution problems in several metropolitan cities and was highlighted in the list of the world's most polluted cities. Additionally, India has become the most populous nation globally, boasting a population of 1.4 billion people, equating to ~18% of the global population, and experiencing an increased rate of consumption of natural resources. Owing to the country's current scenario, various climate mitigation strategies, including nature-based solutions, must be implemented to reduce such impacts and support India's target of achieving the Sustainable Development Goals (SDGs). This review tries to have a holistic understanding of the effects of climate change on different sectors to identify India's challenges in achieving SDG 13 and SDG 11. Finally, it also highlighted the future recommendations for climate change-related research from an Indian perspective.
1 Introduction
India, with its diverse geography, dense population, and intricate socio-economic fabric, is particularly susceptible to the changing climate. Notably, the issue of air pollution has become a prominent concern in India, further compounded by the effects of climate change. Air pollution in India is fueled by diverse sources, including industrial emissions, vehicular pollution, biomass burning, and dust particles ( Sahu and Saxena, 2015 ; Sonwani, 2016 ; Sonwani et al., 2021a , b , c , 2022a ; Hussain and Hoque, 2022b ). As per the World Air Quality Report State of Global Air (SoGA), many booming cities in India rank among the top 15 most polluted cities of the world viz. Delhi (1st rank), Kolkata (2nd rank) and Mumbai (14th rank).
The county has severe air pollution that triggers climate change and vice-versa. Air pollution and climate change, are closely intertwined ( Singh and Yadav, 2021 ; Sonwani et al., 2022b ). Matyssek et al. (2012) , described air pollution as a component of climate change. Anthropogenic emissions play a significant role in causing both air pollution and climate change. In addition to the greenhouse gases (GHGs) such as—carbon dioxide (CO 2 ), methane (CH 4 ), nitrous oxide (N 2 O), water vapor (H 2 O), hydrochlorofluorocarbons (HCFCs), hydrofluorocarbons (HFCs) and Tropospheric ozone (O 3 ), air pollutants such as black carbon (BC), brown carbon (BrC) and aerosol also having significant impact on climate ( Ramanathan and Carmichael, 2008 ; Sonwani et al., 2021a , b , c , 2022a ). Apart from the direct climate impact of rising ground-level ozone, it also indirectly affects the lifespan of other GHGs like methane and causes additional climate impact ( Singh and Yadav, 2021 ). Conversely, climate change influences ozone concentrations through both dynamic and chemical changes in the atmosphere. Moreover, the rising earth's surface temperature causes glaciers' melting and sublimation, causing resulting in more atmospheric water vapor, additionally participating in secondary air pollutant formation causing ecological damage ( Saxena and Sonwani, 2019a ). Thus, we have scientific evidence which proves that air pollution and climate change together impact the composition of the atmosphere and are responsible for environmental damage.
Climate change is an unprecedented global challenge that has far-reaching consequences for countries around the world ( Klingenfeld and Schellnhuber, 2012 ; Haibach and Schneider, 2013 ). The aftermath of climate change in India manifests in various forms, including rising temperatures ( Krishnan et al., 2020 ), altered precipitation patterns ( Behera et al., 2019 ; Sonwani and Kulshrestha, 2019 ), increased regularity and strength of severe weather occurrences ( Hussain and Hoque, 2022a ; Saxena et al., 2022 ); sea-level rise ( Krishnan et al., 2020 ), instance and frequent forest fire incidences ( Jain et al., 2021 ), sever dust storm incidences and draft ( Maji and Sonwani, 2022 ). These impacts permeate critical sectors including agriculture, water resources, public health and biodiversity.
The pervasive effects of climate change in India span across multiple sectors, posing significant obstacles to achieving Sustainable Development Goal 13 (SDG 13) - Climate Action. The bad air quality with changing climate exacerbates one another issue, which is variable atmospheric composition ( D'Amato et al., 2002 ). This not only poses significant risks to respiratory illnesses and cardiovascular diseases ( Ravindra et al., 2019 ; Goel et al., 2021 ; Sonwani et al., 2022a ), but also impairs agricultural productivity ( Wiebe et al., 2019 ), economic loss ( Sathaye et al., 2006 ; Saurabh Sonwani and Vandana Maurya, 2019 ; Parikh and Bhavsar, 2023 ) disrupts vegetation and ecosystems, and hampers sustainable development ( Sonwani et al., 2022b ). The Climate India 2022 report by the Center for Science and Environment (CSE) highlighted that India recorded weather extremes on 22 out of 273 days between January to September 2022 ( Climate India, 2022 ).
Tackling the aftermath of climate change and addressing air pollution necessitates a comprehensive and interdisciplinary approach. Achieving SDG 13 requires adopting efficient strategies to minimize the release of greenhouse gases, smart agricultural practices, promoting renewable energy sources, and enhancing energy efficiency. Also, implementing adaptation strategies, green infrastructure, and environmentally sustainable and socially resilient cities, and efficient waste management approaches are crucial for SDG 11 to minimize the climate change impact ( Zakaria et al., 2020 ). Moreover, sustainable water management ( Xiang et al., 2021 ), air and watershed management and enhancing disaster preparedness with early warning system are also useful to combat changing climate ( Saxena and Sonwani, 2020 ; Hussain and Hoque, 2022a ).
The objective of this review is to highlight the current status and menace of air pollution and climate change and their pervasive effects in India. It also explores the potential solutions and related challenges to achieve SDG 13 ( Climate action ) with special reference to climate change adaptation and mitigation strategies. Furthermore, the impact of the changing climate on SDG 11 (S ustainable Cities and Communities ) was also highlighted in reference to sustainable infrastructure and healthy well-being in the Indian context. A flow diagram summarizing the adverse effects of climate change, and possible strategies for achieving the targeted sustainable development goal has been presented in Figure 1 . This review makes an effort to have a holistic understanding of the effects of climate change (extreme events, warming, etc.) on different sectors (agriculture, health, and communities) so as to identify India's challenges in achieving SDG 13 and SDG 11.
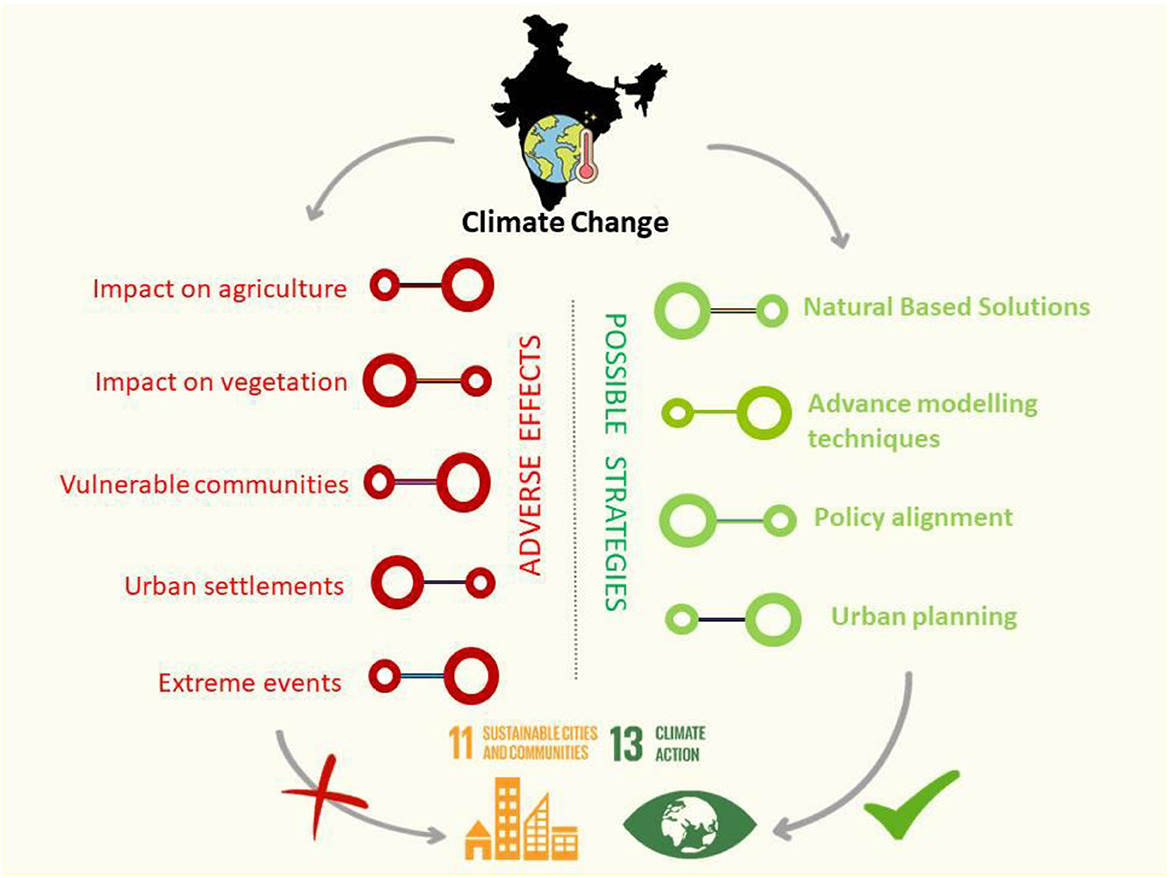
Figure 1 . A schematic representation summarizing the adverse effects of climate change and possible strategies to achieve the selected sustainable goals.
2 Status of climate change in India
The susceptibility of developing countries to the consequences of climate change in comparison to developed countries is now widely recognized. This is due to the fragile ecological environments, high risk to economic systems, and low-income levels of the majority of citizens which limit the ability to adapt ( Panda, 2009 ). The rapid urbanization, alterations in river patterns, the impact of shifting cultivation practices, the spread of erosion, and the progression of desertification are resulting in significant changes in land use. These changes in land use have a direct correlation with the hydrological cycle, as noted by Sreenivasulu and Bhaskar (2010) , and they play a pivotal role in causing extensive transformations within associated ecosystems, as highlighted by Chakraborty (2009) . Developing countries, such as China and India collectively contribute a significant share of global carbon dioxide (CO 2 ) and GHG emissions, mainly due to their growing populations and industrialization ( Ahmed et al., 2022 ; Pathak, 2023 ). Between 1970 and 2021, India experienced a total of 573 disasters related to extreme weather, climate, and water events, resulting in the loss of 138,377 lives, as reported by the Indian Meteorological Department ( IMD Annual Report, 2021 ). India has experienced the highest temperatures and the most prolonged dry spells ever recorded in recent years ( Panda et al., 2017 ) and is now listed in the UN's Global Drought Vulnerability Index with consecutive drought episodes occurring in the major rice-producing states such as UP, Bihar, Jharkhand, and West Bengal ( Amrit et al., 2021 ). Simultaneously, frequent episodes of intense, short-lived downpours resulting in flooding incidents, such as the deluge in Uttarakhand in the year 2013, Kashmir in the year 2014, Kerala in 2018 etc. have been reported ( Akram et al., 2023 ). Recently, India has become the most populous nation globally boasting a population of 1.4 billion people, equating to 17.7% of the global population ( Salunke, 2022 ) which would lead to an increasing carbon footprint. According to a study by Chaturvedi et al. (2012) , the Coupled Model Intercomparison Project Phase 5 (CMIP5) ensemble mean climate results for India in the business-as-usual (RCP6.0–RCP8.5) scenario , the mean warming was predicted to be between 1.7–2°C by 2030 and 3.3–4.8°C by 2080 in comparison to pre-industrial times. Whereas, under the business-as-usual scenario , all-India precipitation is predicted to increase from 4% to 5% by 2030 and from 6%−14% toward the end of the century (2080s).
Due to rapid economic and developmental growth, India is experiencing sector-specific CO 2 emissions from four major sectors Electricity (35.05%), Agriculture (23.18%), Construction/Manufacture (15.92%), and Transport (8.64%) ( Tiseo, 2023a ). While per capita CO 2 emissions in India were relatively low at 1.82 metric tons and significantly below the global average of 4.55 metric tons ( World Bank, 2021 ), the country ranked as the fourth-largest emitter in 2017 due to its substantial population and economic size ( UNEP, 2019 ). Per capita CO 2 emissions in India have shown an increasing trend, rising from 0.39 metric tons in 1970 to a peak of 1.91 metric tons in 2022 ( Tiseo, 2023b ). Furthermore, carbon dioxide emissions stemming from the use of fossil fuels and industrial activities in India surged by 6.5% in 2022, setting a new record at 2.7 billion metric tons (GtCO 2 ) ( Tiseo, 2023c ). In terms of annual anthropogenic emissions, India is estimated to release 47,000 million metric tons of CO 2 , 570 million metric tons of CCl 4 , and 9 million metric tons of N 2 O ( Basha and Reddy, 2022 ). Emissions of a few pollutants in India were estimated to be 2.144 tons/capita of GHG emissions, 69.4 kg/capita of CO emission, 20.36 kg/capita of NOx emission, and 9.51 kg/capita of SOx emission ( OECD, 2023 ). Additionally, climate change-led disasters like forest fires add more concentration to the pollutants. Each year, the world sees ~4.3–5.5 GtCO 2 e/year emissions caused by changes in land use and land cover ( Sannigrahi et al., 2020 ). Between 2003 and 2017, a significant number of 520,861 forest fires were identified across the diverse forest landscapes of India ( Sannigrahi et al., 2020 ). For instance, the Uttarakhand Forest fire caused an increase of 52% in CO, 52% in NOx, and 11% in O 3 during the High Fire Activity Period (HFAP).
2.1 Temperature and rainfall anomaly
The mean temperature across India in 2021 averaged 21.43°C, ranking as the third-highest on record since 1901, following the years 2016 (21.8°C) and 2009 (21.59°C) ( IMD Annual Report, 2021 ). In Figures 2A , B , we can observe the deviations from the average seasonal maximum and minimum temperatures. Figure 3 highlighted the time series of average mean temperature for the post monsoon season, over India for a period from 1971–2021. Over most regions of the country, the maximum temperature exceeded the normal values, with exceptions in certain areas of northwest India, eastern and northeastern India, central India and southern peninsular India. Particularly in some areas, such as Assam and Meghalaya, Nagaland, Manipur, Mizoram, Tripura, Himachal Pradesh and Saurashtra and Kutch, experienced maximum temperature anomalies exceeding 2°C. Similarly, when looking at the minimum temperatures, they were generally higher than the typical values for most parts of the country, except for specific regions in northwest India, central India, southern peninsular India, and Lakshadweep. Notably, some areas, including northern Saurashtra and Kutch, central Maharashtra, Bihar, North Interior Karnataka, and southern Kerala and Mahe, witnessed minimum temperature anomalies surpassing 2°C. Conversely, certain areas like Delhi, West Uttar Pradesh, Haryana, Chandigarh, East Rajasthan, southern Madhya Pradesh, Chhattisgarh, Vidarbha, Andhra Pradesh, Telangana, and South Interior Karnataka experienced minimum temperature anomalies lower than −1°C ( IMD Annual Report, 2021 ). There has been visible evidence of altered precipitation patterns over the country. In some regions of Tamil Nadu, Puducherry, Karaikal, Kerala, Mahe, and Lakshadweep, the rainfall anomaly exceeded 100 mm. Conversely, there were areas in Arunachal Pradesh, Assam, Meghalaya, Uttarakhand, Punjab, Himachal Pradesh, and the western parts of Jammu and Kashmir and Ladakh where the negative rainfall anomaly was greater than 50 mm. Furthermore, in parts of Arunachal Pradesh, Uttarakhand, Punjab, Himachal Pradesh, and Jammu and Kashmir, the negative rainfall anomaly exceeded 75 mm ( Figures 4 , 5 ).
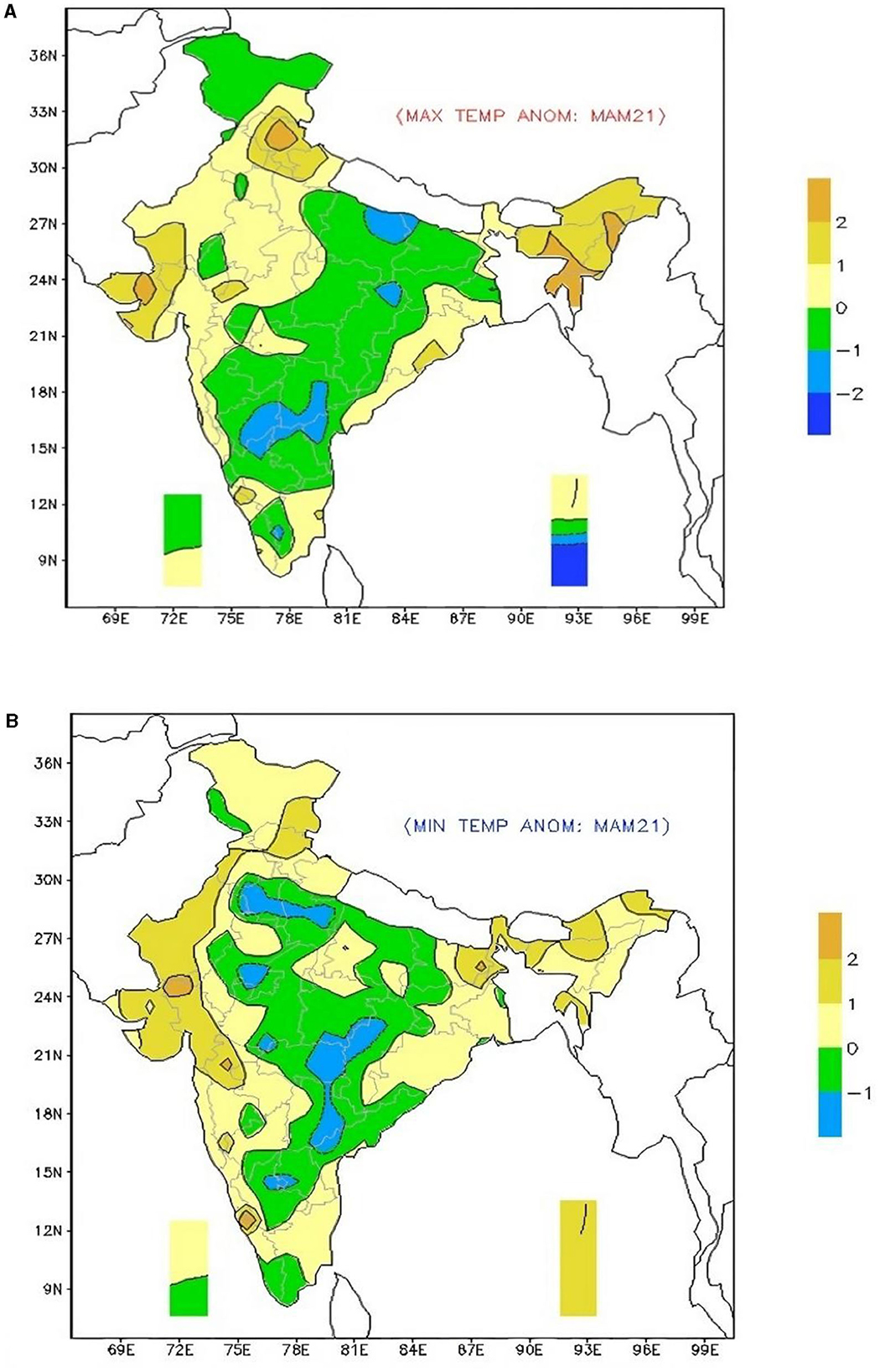
Figure 2 . Mean seasonal temperature anomalies (°C) (A) maximum (B) minimum (source: IMD Annual Report, 2021 ).
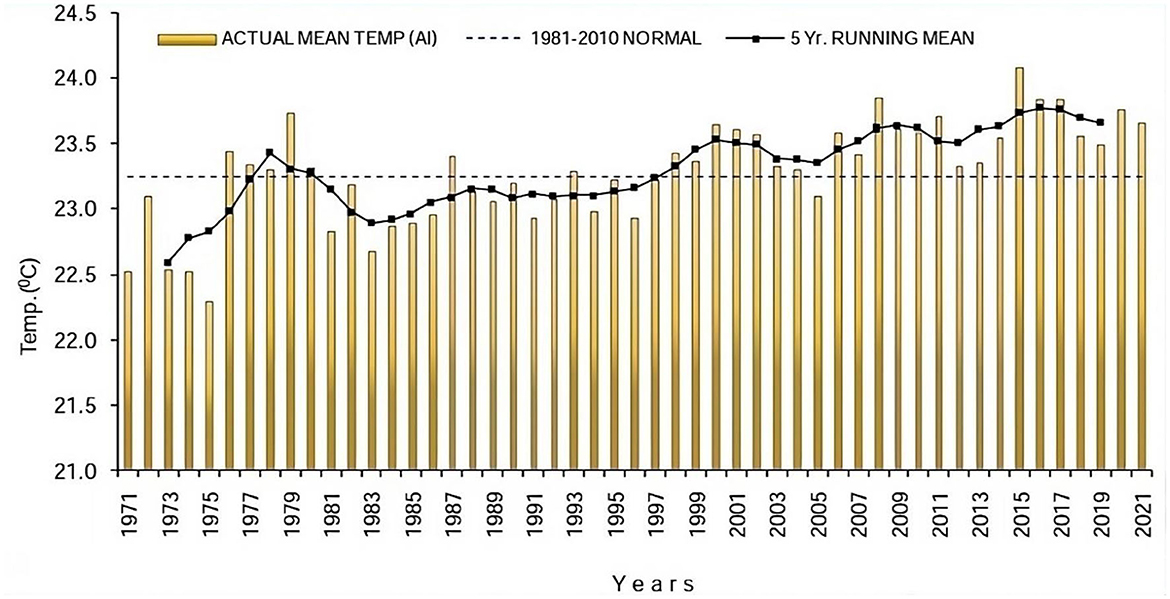
Figure 3 . Time series of mean temperature averaged over India (vertical bars) and 5 year running mean (continuous line) for the post monsoon season (1971–2021) (source: IMD Annual Report, 2021 ).
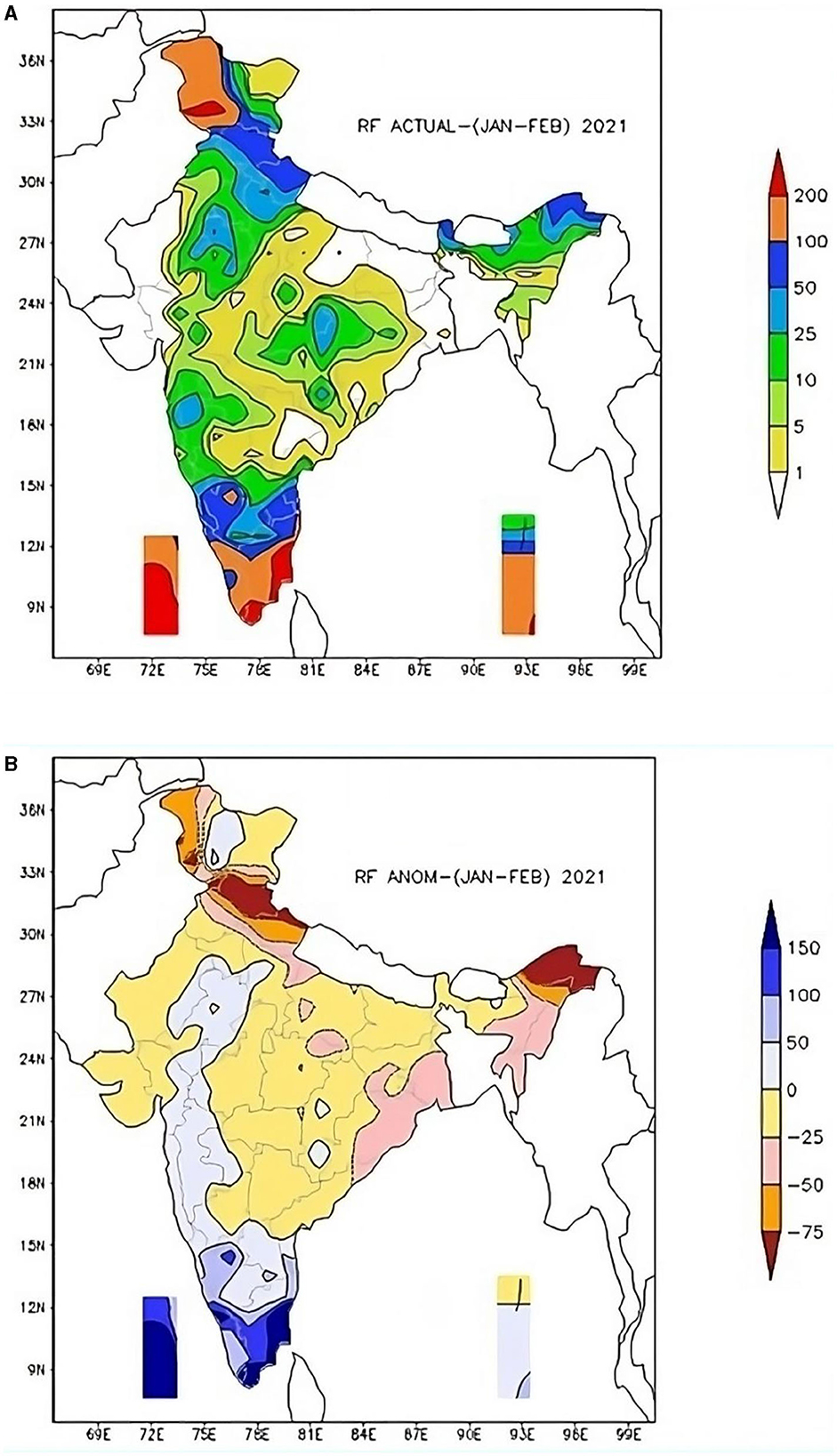
Figure 4 . Spatial pattern of (A) seasonal rainfall winter (January–February) (B) seasonal rainfall anomaly (mm) (source: IMD Annual Report, 2021 ).
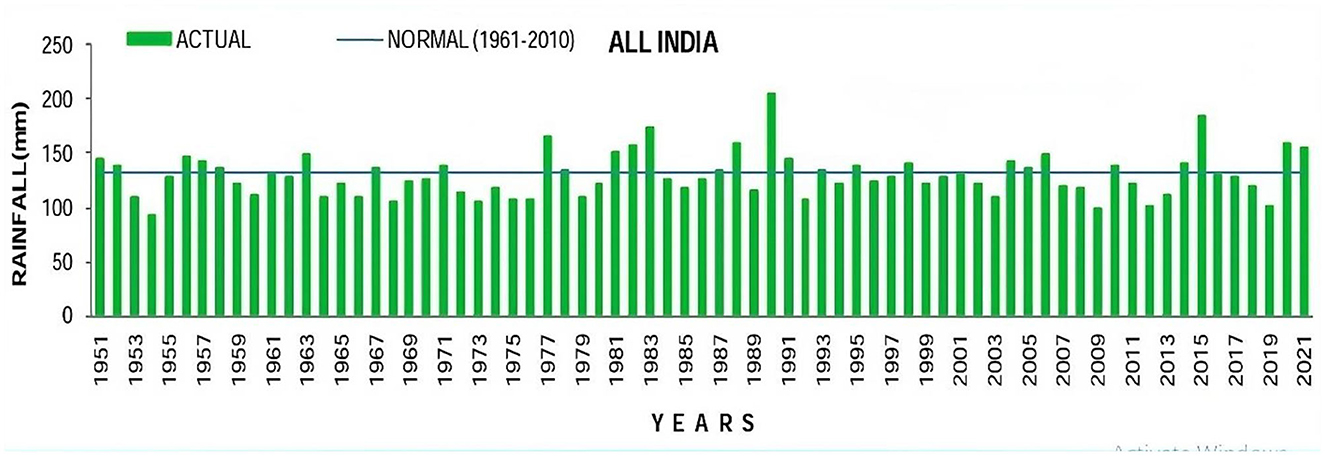
Figure 5 . Seasonal rainfall over the country as a whole for the period 1951–2021 (source: IMD Annual Report, 2021 ).
2.2 Climate change transitions and projections against climate variables in India
Figure 6 shows India's transitions in relation to climate change. It mentioned the different initiatives related to the summits, agreements, rectifications, national action plans, and launch and amendments of any acts relevant to climate change and mitigation.
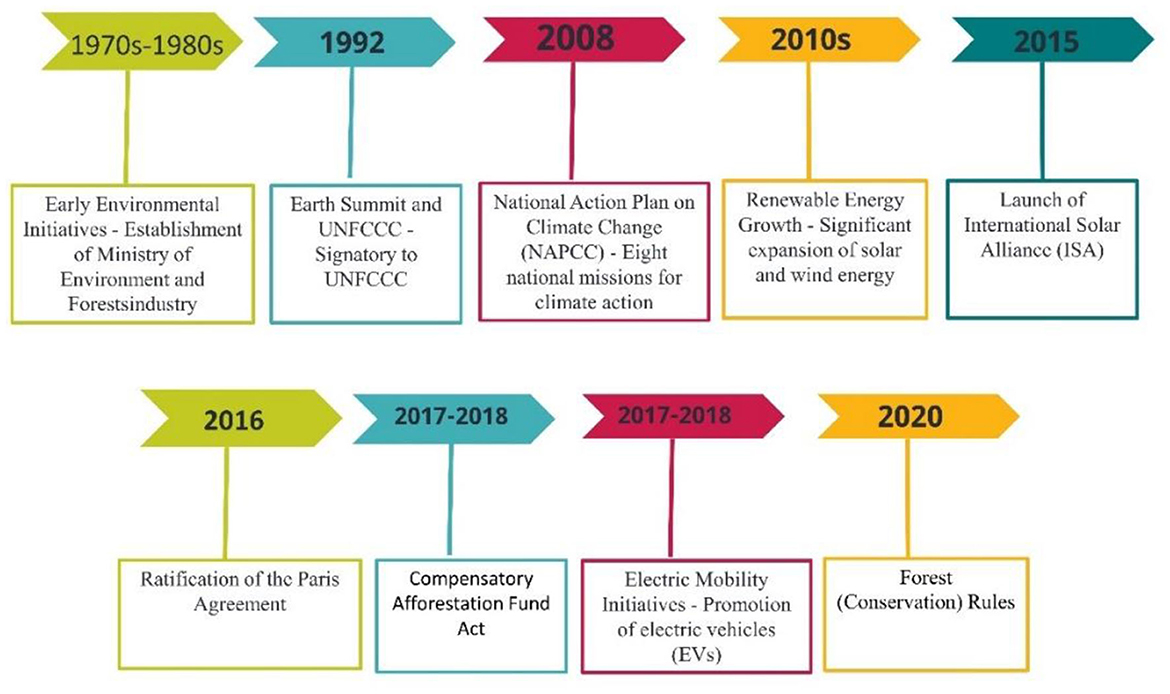
Figure 6 . Schematic diagram of timeline for India's transition in climate change.
The repercussions of climate change, encompassing escalating temperatures, acidification of oceans, and rising sea levels, have yielded adverse consequences for both essential services and the livelihoods of those reliant upon them. Since the 20th century, Asia has witnessed a consistent rise in surface air temperatures, resulting in significant concerns for social and economic stability ( Ren et al., 2023 ). This triggers an increased likelihood of heat waves, alterations of the monsoon patterns, frequent flood occurrences in regions experiencing monsoons, and the melting of glaciers in the Hindu Kush Himalaya area ( United Nations Environment Programme, 2009 ).
India, as a developing nation, contributes to around 3.2% of the total global Gross Domestic Product (GDP). It's worth noting that India is home to nearly 17.7% of the world's population and is responsible for ~6.8% of the world's carbon emissions, placing it fifth in terms of geopolitical risk rankings ( Adebayo et al., 2023 ). Significantly, a 10% increase in Economic Policy Uncertainty in India could have adverse effects on the economy, potentially hindering the green economy by reducing it by 0.535% ( Liang and Qamruzzaman, 2022 ). The danger of irreversible loss extends to vital marine and coastal ecosystems, including coral reefs, tidal marshes, seagrass meadows, and plankton communities ( UN, 2022 ). Meanwhile, mangroves in India remain under continuous threat from pollution, conversion for aquaculture and agriculture, as well as challenges related to climate such as sea level rise and coastal erosion ( Akram et al., 2023 ). These risks also drastically intensify, for example, global warming exceeds a 2°C rise in temperature and calls for fluctuations in the climate system. In India, the hotter summer climate has led to a surge in demand for cooling, compounding the situation due to rapid population growth ( Hoegh-Guldberg et al., 2018 ). These climatic factors have introduced water stress affecting both water supply and demand. Scarce precipitation further impacts water supply demands, prompting the increased use of energy-intensive techniques like desalination and underground water pumping ( IPCC, 2023 ).
Transboundary river basins like the Indus and Ganges in the mid-21st century are predicted to face severe water scarcity. India, in particular, faces an elevated risk of experiencing increased drought conditions ranging from 5% to 20% by the close of this century ( IPCC, 2023 ). Additionally, the security of local and downstream communities is threatened by Glacier Lake Outburst Floods (GLOFs). Moreover, the persistence of rising temperatures, shifting precipitation patterns, and climate extremes such as heat waves, droughts, and typhoons will maintain their role as pivotal vulnerability factors, significantly shaping agricultural productivity in Asia ( IPCC, 2023 ). Projections for the agricultural and food industry include notable declines in fisheries, aquaculture, and agriculture yield, specifically in South Asia. In India, rice production may decline by 10%−30% and maize production by 25%−70%, considering a temperature increase in the range of 1°C−4°C. In this context, Krishnan et al. (2020) published a report by the Ministry of Earth Sciences (MoES), Government of India where past and projected changes in the country due to climate change have been discussed in detail ( Table 1 ).
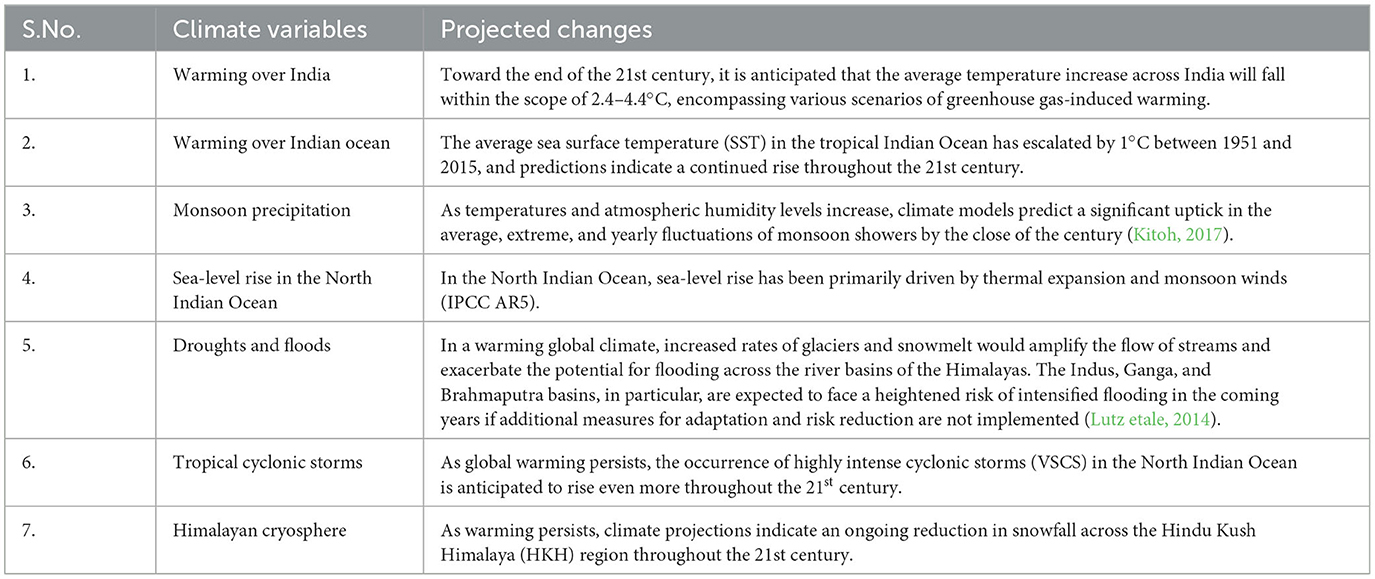
Table 1 . Table listing projected changes against different climate variables in India (adapted from Krishnan et al., 2020 ).
3 Climate change impacts
3.1 impact on forest.
Climate change exerts an impact on various natural disruptions that jeopardize the wellbeing of forests. These encompass insect infestations, the intrusion of non-native species, wildfires, and severe storms. Certain disturbances, such as wildfires, occur abruptly. The consequences of climate change on forests carry significant implications for those individuals whose livelihoods rely on forest resources. India, being a mega-biodiversity nation, dedicates over one-fifth of its land to forests. In a country with almost 173,000 villages designated as forest villages, there is a substantial reliance of communities on these forest resources ( Gopalakrishnan et al., 2011 ). The effects of climate change on India's vegetation are region-specific. For instance, the Himalayan region is particularly vulnerable to the loss of alpine meadows and the upward shift of tree-line due to rising temperatures ( Manish et al., 2016 ). The coastal mangrove forests of India also face detrimental effects, including increased saltwater intrusion and decreased productivity, due to the effects of climate change ( Semba et al., 2022 ).
Climate plays a pivotal role in shaping vegetation patterns on a global scale and exerts a substantial impact on the arrangement, composition, and ecological dynamics of forests ( Kirschbaum et al., 1996 ). The 2022 IUFRO hybrid meeting held in Vienna, Austria was themed “Forests in a Volatile World—Global Collaboration to Sustain Forests and Their Societal Benefits”. The annual report evaluates the world's progress in minimizing emissions from deforestation and deterioration of forested areas. (REDD+) and was prepared by IUFRO's Global Forest Expert Panels (GFEP) Programme (this full line should be deleted). A key takeaway from their report is that although REDD+ has served as a convenient framework for various activities focused on curbing deforestation and forest degradation, the intricate complexities between forests, land use, and climate still remain an issue ( IUFRO Report, 2020 ). In a warmer world, the forests currently play a vital role as carbon sinks and could be entirely eroded. Land ecosystems may transition from being a net carbon dioxide absorber to becoming a significant source of carbon dioxide emissions ( Seppälä et al., 2009 ).
In India, air pollution and climate change have profound effects on vegetation, posing significant challenges to the country's ecosystems and terrestrial productivity. Air pollutants can harm the health of trees, leading to leaf discoloration, reduced growth, and even tree mortality. Climate change-induced factors like rising temperatures and alterations in rainfall distribution, and altered pest and disease dynamics further stress forest ecosystems, potentially leading to forest decline and loss of biodiversity ( Saxena and Sonwani, 2019b ; Saxena and Srivastava, 2020 ; Sonwani and Saxena, 2022 ; Sonwani and Shukla, 2022 ; Sonwani et al., 2022a ).
According to a study by Chaturvedi et al. (2011) , which utilized the Regional Climate Model of the Hadley Center (HadRM3) and the dynamic global vegetation model IBIS, projections were made for climate change scenarios. The study highlights that the upper Himalayas, northern and central areas of the Western Ghats, and certain regions of central India are particularly susceptible to the expected effects of climate change. On the other hand, the assessment suggests that the forests in the northeastern part of India exhibit better capacity to adapt or withstand the projected changes in climate.
One of the notable impacts of climate change on vegetation is the shifting distribution and composition of plant species. As temperatures increase, certain plants may struggle to survive in their existing habitats and gradually migrate to more suitable regions ( Chauhan et al., 2022 ). This phenomenon, known as “shifting vegetation belts”, Has the potential to result in alterations in the functioning of ecosystems, affecting the interactions between plants, animals, and their environment ( Sonwani et al., 2022a ). Another evident impact is the alteration of phenological patterns in plants ( Corlett and Lafrankie, 1998 ; Workie and Debella, 2018 ) such as changes in flowering and fruiting seasons. Climate change-derived shifts in temperature and rainfall patterns can disrupt the synchronicity among plants and their pollinating or seed-dispersing agents, potentially leading to mismatches in these critical ecological relationships. Additionally, the study conducted by Ranjan and Gorai (2022) examined the start-of-season (SOS) and end-of-season (EOS) trends for various vegetation types. During the period of 2001–2010, most vegetation types (excluding winter snow vegetation and mixed forest) exhibited a delay in both SOS and EOS. The delay occurred at a rate ranging from 0.009 to 0.29 days per year for SOS and 0.03 to 0.33 days per year for EOS. Conversely, from 2010 to 2019, the EOS and SOS of all vegetation types (except mixed forest) displayed an advancing trend. The SOS advanced at a rate of 0.06 to 0.24 days per year, while the EOS preponed at a rate of 0.17–0.23 days per year. These findings indicate significant changes in the timing of the period of growth for various types of vegetation. Such alterations can have implications for plant growth, phenology, and ecosystem dynamics ( Shrestha, 2019 ). Furthermore, climate change threatens the overall productivity and health of vegetation in India ( Upgupta et al., 2015 ; Ahmad et al., 2018 ). Increased heat waves and droughts can induce water stress on plants ( Marchin et al., 2022 ), impacting their growth, productivity, and survival. Additionally, rising temperatures generate a conducive environment for the rapid increase of pests and diseases ( Shrestha, 2019 ; Zayan, 2019 ), further endangering the health and resilience of plant populations.
The study by Haughan et al. (2022) on the impact of climate change on Indian forests reveals a significant rise in annual forest loss during the 17-year period from 2001 to 2018, with the Northeast region experiencing the highest proportion of this loss. This increase in forest loss is attributed to changing trends in temperature and precipitation over time. Their study raises concern about climate velocities recorded in the country, reaching 97 km/year. They underscore the importance of comprehending the unique regional and seasonal connections between climatic conditions and forest distribution to effectively conserve the remaining forests as climate change continues to accelerate.
3.2 Impact on agriculture
Agriculture, being a vast sector highly sensitive to weather conditions, is particularly vulnerable to the impacts of climate change, resulting in significant economic consequences. Variations in climatic factors like temperature and rainfall have a profound effect on crop yields. The influence of rising temperatures, changes in precipitation, and increased CO 2 levels vary depending on the specific crop, location, and the extent of these alterations ( Malhi et al., 2021 ). Additionally, regions with higher humidity and warmer climates tend to face greater challenges from insect pests and diseases ( Malhi et al., 2021 ). To meet the food and nutritional demands of the global population by 2050, it is imperative to achieve a 60% increase in worldwide agricultural production from 2005/2007 levels, with developing countries aiming for a 77% increase and developed nations targeting a 24% rise ( Alexandratos and Bruinsma, 2012 ). Any loss in crop yields can potentially drive up food prices and have a substantial impact on global agricultural well-being, potentially resulting in a 0.3% annual decrease in global GDP by 2100 ( Stevanovic et al., 2016 ). Recent evidence suggests that approximately 9% of cropland expansion in developing countries over the last two decades can be attributed to dry anomalies, as farmers expand their agricultural areas to compensate for yield losses ( Zaveri et al., 2020 ).
Guntukula (2020) conducted an analysis using annual time-series data spanning 58 years (1961–2017) to examine the impact of climatic variables, specifically rainfall, maximum temperature, and minimum temperature, on the yields of seven major crops in the region viz. rice, wheat, pulses, rapeseeds and mustard, cotton, sugarcane, and groundnut in India. The study observed that rainfall, and maximum and minimum temperatures, have a substantial effect on crop productivity. The specific impact of these variables varied across different crops and regions. A sensitivity analysis using CERES (crop estimation through resources and environmental synthesis) has also shown that wheat and rice yields in northwest India have the potential to increase by 28% and 15%, respectively, at double the levels of CO 2 ; however, the increased thermal stress due to elevated level of temperatures associated with high CO 2 nearly cancels out the positive impact ( Malhi et al., 2021 ).
India has already witnessed adverse impacts from recent climate patterns, which are attributed to emissions on a worldwide scale of both long-lived greenhouse gases (LLGHGs) and other short-lived climate pollutants (SLCPs) particularly, tropospheric ozone and black carbon ( Burney and Ramanathan, 2014 ). These pollutants not only have indirect effects on crop yields through climate changes but also exert direct effects. Burney and Ramanathan (2014) investigated the combined effects of climate change, the direct consequences of short-lived climate pollutants (SLCPs), and air pollutants on wheat and rice crop yields in India spanning the years from 1980 to 2010. Their statistical model revealed that, on average across the country, wheat yields and rice yields in 2010 were up to 36% and 20% lower respectively, than in a scenario where climate change had not occurred. Remarkably, the upper-bound estimates suggested that 90% of these yield losses can be associated with the direct effects of SLCPs. This finding underscores the potential gains that could be achieved by addressing regional air pollution. By mitigating the direct climate change effects of LLGHGs through concerted efforts to reduce SLCP emissions, we could counter anticipated future yield losses and safeguard agricultural productivity in India.
Gupta et al. (2017) employed regression analysis using information from 208 districts to investigate the influence of temperature and aerosol pollution, on wheat yields in India from 1981 to 2009. Their results showed that an increase of 1°C in the daily average maximum and minimum temperatures corresponds to a decrease in yields by ~2–4% each. They combined the estimated impacts of climate change on productivity along with aerosol pollution. The results revealed that a one-standard-deviation reduction in aerosol optical depth (AOD) is projected to enhance yields by ~4.8%. These findings suggest that by mitigating regional air pollution and addressing global warming in the coming decades, it is possible to counter the losses in wheat yields.
3.3 Impact on human health
The amalgamation of climate change and air pollution has profound and multifaceted implications for human health ( Kumar et al., 2019 ). The intricate interplay between climate change and air quality suggests that future strategies aimed at addressing these interconnected issues will gain advantages from increased coordination ( Kinney, 2018 ). Young children, senior citizens, and pregnant women are particularly susceptible to the health risks associated with air pollution, as their respiratory and cardiovascular systems are more fragile and prone to damage ( WHO, 2016 , 2021 ; Sonwani et al., 2021a , b , c ). Young children are particularly susceptible because they have faster breathing rates in comparison to adults ( Sharma and Kumar, 2018 , 2020 ), the breathing rate of children per unit of body weight is nearly double compared to that of adults ( Harrison and Yin, 2000 ; Ginsberg et al., 2005 ; Heal et al., 2012 ) and their organ systems are in a phase of rapid growth and development. These effects have been discussed in detail in previous studies by Sharma and Kumar (2018 , 2020) .
People inhabiting in urban ecosystems are fragile to health impacts owing to high temperature and extreme pollution levels. Research has consistently shown that city temperatures tend to be higher than those in open rural areas, giving rise to the phenomenon known as the urban heat island (UHI) ( Choudhury et al., 2023 ). In recent decades, many major global cities have undergone significant changes in land use and land cover, with far-reaching effects on their urban ecosystems. These changes have resulted in issues such as increased air pollution, traffic congestion, land degradation, and the emergence of urban heat islands (UHIs) ( Yan et al., 2016 ; Liu et al., 2020 ). The connection between urban heat islands and climate change is a consequence of urbanization, industrialization and other human-induced factors. In developing countries such as India, where air quality deteriorates below acceptable levels ( WHO, 2021 ), citizens from poor socioeconomic backgrounds are disproportionately affected due to health risks from air pollution ( Kumar et al., 2015 ). The urban communities of our country are also subjected to extreme pollution levels and climate change impacts due to the UHI effect. According to a study conducted in Delhi ( Singh et al., 2022 ) in the year 2000, the minimum temperature in Delhi was 23.2°C, and the maximum was 34.85°C. By 2010, these temperatures had increased to 26.31°C and 39.92°C, and in 2020, they further rose to 31.7°C and 44.74°C. This significant temperature rise over the years illustrates the UHI effect. Moreover, the normalized differential vegetation index (NDVI) has revealed a notable reduction in non-vegetated areas, decreasing from 82% to 62% due to rapid urbanization and changes in land use and land cover over the past two decades. These biophysical changes in the city's landscape clearly indicate that NDVI and land use/land cover modifications are the primary drivers behind the development of urban heat islands within the city. This worsens existing health inequalities and highlights the pressing demand for focused interventions to safeguard such vulnerable populations ( Mathiarasan and Hüls, 2021 ). One crucial finding from epidemiological research is that babies transported in prams are highly vulnerable to air pollution. They breathe at lower heights, typically within 1 meter from the ground level and in close proximity to vehicle tailpipes emitting harmful pollutants ( Sharma and Kumar, 2018 , 2020 ) raises concerns about their long-term health and emphasizes the urgency of addressing air quality in urban environments.
To mitigate air pollution exposures, dedicated efforts are needed to quantify the obstacles and opportunities to effectively utilize scientific knowledge for managing air quality while accounting the diverse stakeholder's perspective ( Muñoz-Pizza et al., 2022 ). Many of the researchers identified different COVID-19 lockdown phases as a proxy solution for air pollution mitigation, that may be implemented in future for such air pollution and climate benefits ( Venter et al., 2020 ; Goel et al., 2021 ). For the effective implementation of such strategies requires effective atmospheric monitoring, emissions inventories, and compilation of health-related datasets particularly for developing countries. Further, to derive the necessary shift from an expert-based to a dialogue-based process will need dedicated attempts from multiple stakeholders including scientists, public policy makers, governors, and the citizens ( Green et al., 2000 ). Deploying green infrastructure, promoting sustainable transport fuels with low life-cycle sustainability impacts, and improving public transportation can significantly reduce air pollution levels and improve air quality ( Sharma, 2016 ; Abhijith et al., 2017 ; Abhijith and Kumar, 2019 ; Kumar et al., 2019 ). Moreover, community engagement to sensitize local citizens and policies targeted at reducing individual exposure to air pollution may play a pivotal role in safeguarding health ( Kumar et al., 2015 ).
Tackling the health effects of climate change requires a universal perspective. A large share of responsibility of minimizing climate change lies with developed countries because they have historically emitted greenhouse gases. Still, its consequences are often felt most severely by poor people in developing countries ( Kumar et al., 2015 ; Perera, 2018 ). As a result of this environmental injustice, it demonstrates the moral imperative for collective action to mitigate climate change, promote sustainable practices, and ensure that the burden of climate change does not fall disproportionately on the most vulnerable populations ( Perera, 2017 , 2018 ). In a nutshell, there should be a greater focus on environmental justice and equitable global responsibility to minimize air pollution exposure and address climate change impacts.
4 Climate change related extreme events in India
The threat of air pollution in India has risen, with its impact exacerbated by the influence of climate change. Under this section we list few among many extreme events reported in India that was triggered by climate change.
In December, 2015, Chennai experienced extreme one-day rainfall causing widespread flooding. The likely cause to be detected was aerosols counteracting with greenhouse gases ( van Oldenborgh et al., 2016 ). Anthropogenic warming was also blamed for warm mean surface temperatures observed in 2015 according to CMIP5 simulations ( Kam et al., 2016 ; Nanditha et al., 2020 ). Climate change was attributed as the cause for the total rainfall in northern India during June 2013 ( Singh et al., 2014 ). Indian states situated in the flood-prone area of the Ganges-Brahmaputra-Meghna basin, encounters a series of unfavorable climate occurrences notably, annual floods. A study focused on the flood catastrophe that took place in the Ganga-Brahmaputra basin in 2020, impacting the regions of Bihar, West Bengal, and Assam during July, August, and September. They derived the flood extent using Google Earth Engine (GEE) and data from Sentinel-1A Synthetic Aperture Radar (SAR). The combined extent of floodwater inundation between July and September was calculated to be highest in Bihar (20,837 km 2 ) followed by West Bengal (17,307.1 km 2 ), and Assam (13,460.1 km 2 ) ( Pandey et al., 2022 ). This event was attributed to global warming, sea level rise and climate change catalyzed glacier melting ( Barman and Bhattacharjya, 2015 ; Janes et al., 2019 ; Loukas et al., 2021 ).
As a consequence of climate change induced warming, it was predicted that a 10–12% increase in precipitation and a sea level rise of approximately 50 cm could result in the submergence of numerous coastal cities in India, including Mumbai, Chennai, Goa, Kochi, Puducherry, Daman and Diu. This, in turn, would place an estimated 35–50 million Indians at risk of chronic flooding, potentially leading to loss of life (National Maritime Foundation, NMF).
4.2 Storms and cyclone
As a results of climate impact, several coastal cities experienced the high incidences of cyclonic events. Repeatedly, storms and cyclones have wrought havoc upon numerous villages in the coastal state of Odisha, which is frequently susceptible to tropical cyclones. Consequently, residents have been compelled to seek new habitation. A noticeable surge in the frequency of storms, and cyclones has been documented over the past few decades when compared to historical records ( Subramanian et al., 2023 ). Sand and dust storms become very common in the North and West Indian region and have a variety of adverse impact on the environment. The principal consequences of these incidents encompass alterations in the atmospheric radiation balance, changes in regional precipitation patterns, and fluctuations in hurricane activity. Furthermore, dust storm events contribute to heightened concentrations of fine particulate matter in the atmosphere, which have been linked to adverse health effects such as premature mortality, cardiovascular issues, respiratory problems, lung cancer, and severe respiratory tract infections ( Maji and Sonwani, 2022 ).
4.3 Forest fire
Globally it is recognized that ~80% of forest fires are anthropogenic in nature ( FAO, 2007 ). In India, ~50% of the forest areas are classified as fire prone and > 95% of forest fires are of anthropogenic origin ( Satendra and Kaushik, 2014 ). The occurrence, frequency, and intensity of forest fires is directly linked to changing climate conditions such as warmer temperature, precipitation deficits, increased number of dry days, and El Niño-Southern Oscillation (ENSO) events [ National Oceanic and Atmospheric Administration (NOAA), 2021 ] that can lead to an increase in fire incidences ( Nurdiati et al., 2022 ). It has also been recently investigated that, due to changing climate increasing the forest fire intensity, incidences and frequency increased by several fold in central Indian region, such increase was observed not only in the fire prone season, but also in the rest of the year ( Jain et al., 2021 ).
4.4 Drought
Climate change also cause variability in precipitation, soil moisture, and surface water, as well as excessive groundwater extraction, resulting in draft conditions ( Mukherjee et al., 2018 ). According to Roy et al. (2022) , study in which the Drought Early Warning System (DEWS), a system that monitors drought conditions in real-time was used, estimated approximately 21.06% of India's landmass that experienced conditions similar to a drought. This represents a significant increase of 62% compared to the drought-affected area from the same period in the previous year, which was only 7.86%.
4.5 Heat waves and cold waves
Another study focusing on the projected heat waves in South Indian cities using CMIP predicted severe heat waves in the region ( Nandi and Swain, 2022 ). Moreover, other research highlights extreme heat events (EHE) in Ahmedabad, it experienced severe heat waves in 2010 and 2016, recorded temperatures of 50°C (122°F) ( Patel et al., 2022 ). As far as cold waves in India concerned, the north, north-west, central, east India, north-east Peninsula, Jammu and Kashmir falls in the Core Cold Wave Zone (CCZ). The El-Niño event in India hampers the CW activities. Significant increasing trends in the heat waves days and noticeable reductions in the frequency of cold wave days, aligning with similar trends was observed in numerous regions worldwide ( Pai and Nair, 2022 ).
5 India's urban transformation and climate change
Urban systems play a crucial role in achieving significant reductions in emissions and promoting the establishment of communities with the capacity to withstand and adapt to the effects of climate change. Cities need to consider various factors for both adaptation and mitigation, such as assessing climate addressing impacts and vulnerabilities by means of climate-related services in the planning and design of infrastructure and settlements. Strategies like compact urban planning, integrating jobs and housing, promoting public transportation and active modes of mobility like walking and cycling, as well as efficient construction, retrofitting, and energy-efficient use of buildings, contribute to these goals ( Chen et al., 2008 ; Barton and Tsourou, 2013 ; Amado et al., 2016 ; Bibri et al., 2020 ; Verma et al., 2021 ).
Initially, concerns about climate change impacts in modern India primarily revolved around vulnerabilities to the many climate-related risks. This has led to initial efforts focusing on adaptation measures. The urban transformation in India is influenced by different aspects including population growth, changes in land use, and industrialization, that are multiplied by the impacts of climate change ( Lele et al., 2018 ; Sonwani et al., 2021a , b , c ). The diversity in climate risks across different urban areas in India is a reflection of local geographical and climatic conditions ( Khosla and Bhardwaj, 2019 ). Hence, adaptation strategies need to be tailored to specific local contexts. Furthermore, environmental challenges like air pollution, dwindling green spaces, and water scarcity intersect with the effects of climate change ( Ranjan and Narain, 2012 ; Dholakia and Garg, 2018 ; Gurjar et al., 2018 ).
India's role in these discussions is pivotal, given its projected substantial urban growth in the decades to come ( United Nations Environment Programme, 2021 ). However, many Indian cities are still struggling with foundational issues like physical development, poverty, and environmental degradation. Integrating climate change considerations alongside these basic challenges poses a significant hurdle ( Ürge-Vorsatz et al., 2018 ). The way these cities are constructed and expanded will shape consumption patterns, production practices, and subjected to climate-related risks over decades to come. Many cities lack adequate services, with inconsistent and inadequate access to essentials like water, sanitation, and drainage. A considerable proportion of urban dwellers live in inadequate living conditions, often termed “slums”. The local environment's health, air, water quality and solid waste management, is severely compromised and ranks among the poorest at global level ( Karak et al., 2012 ; Nixon et al., 2013 ; Singh et al., 2013 ). There are some India's strategies for dealing with multifaceted challenges like urbanization and climate change in terms of accessibility, inclusivity, and livelihoods and this emphasizes the urgency of decisions that can establish sustainable, low-carbon, and resilient modern societies ( Creutzig et al., 2016 ; Ürge-Vorsatz et al., 2018 ).
The future path that urban India takes in responding to climate change depends on how effectively regional development and climate objectives are interconnected, combined, and given priority. The way urban areas are built over the next 10 years will significantly influence the consumption habits of the majority of Indians for the rest of the century. Implementing a well-coordinated urban climate strategy that spans different levels of government, taking into account the expansive nature urban layouts of India, and seizing the opportunity to establish sustainable infrastructures with reduced consumption, could play a pivotal role in shaping India's development trajectory. In recent years, urban areas have undergone significant societal and technological transformations that include a noticeable shift toward adopting low-carbon lifestyles ( Roy et al., 2018 ). This shift was made possible by national-level policies and programs influenced by increasing research on modern day climate change. These policies encourage cities to embrace environmentally friendly practices. For example, initiatives like the National Mission on Sustainable Habitat, Smart Cities Mission, Solar City Program, and Green Urban Transport Mission together promote the implementation of rooftop solar panels, energy-efficient practices, public transportation, pedestrian pathways, separate lane for bikes, and building energy standards ( Rajasekar et al., 2018 ; Taraporevala, 2018 ). Concurrently, efforts to enhance drain infrastructure under the Atal Mission for Rejuvenation and Urban Transformation are focused on improving urban climate resilience ( Tewar et al., 2015 ). These localized and sector-specific measures for adaptation and mitigation, along with the associated literature, indicate an increasing recognition and a movement toward climate-focused strategies in urban India.
The initial steps taken for adaptation in urban India were led by two global networks: the Asian Cities Climate Change Resilience Network (ACCCRN) and ICLEI—Local Governments for Sustainability ( Fisher, 2014 ; Khosla and Bhardwaj, 2019 ). The strategies employed for enhancing adaptation and resilience varied based on geographical locations, influenced by local vulnerabilities, risks, and existing infrastructure shortcomings ( International Council of Local Environmental Initiatives-South Asia, 2014 ; UNDP, 2014 ; Yenneti et al., 2016 ; Dhyani et al., 2022 ).
Singh et al. (2021) , studied the climate change approach adopted in some major Indian cities. Out of the 53 cities analyzed, each having a population exceeding 1 million, 38 cities have documented their efforts in either devising or executing strategies to mitigate the impacts of climate change. Additionally, 27 of these cities have outlined plans and, in some cases, already initiated actions geared toward adapting to climate challenges. The range of mitigation measures undertaken is extensive, spanning from the establishment of solar city initiatives in places like Chandigarh and Srinagar, to the development of blueprints for low carbon societies, as seen in Bhopal. Furthermore, more modest interventions have been integrated into smart city projects, encompassing elements like biogas installations and structures powered by solar energy. Among the subset of 27 cities prioritizing adaptation, a diverse array of approaches has been adopted. Some cities have placed an emphasis on systemic enhancements, manifesting in actions rooted in ecosystem preservation, such as the formulation of coastal zone management plans to oversee developmental activities along coastal regions, observed in cities like Mumbai and Chennai. Others have embraced wider nature-based solutions, such as the “Cities4forests” initiative in Kochi, which aims to safeguard, oversee, rehabilitate, and foster green spaces. Moreover, a distinct focus on managing risks is evident in certain cities, with initiatives including action plans to counter the effects of extreme heat, notable examples being Ahmedabad, Hyderabad, and Nagpur. Additionally, the widespread integration of cool roof technologies, notably in Ahmedabad, Indore, and Surat, forms another facet of this risk-focused approach.
6 Vulnerable communities
Climate change is expected to worsen existing disparities related to social factors like caste and gender ( Saini et al., 2015 ), as well as economic aspects like class and geographic location ( Ranjan and Narain, 2012 ). To call out on specific communities, climate change will particularly impact marginalized groups like the urban poor individuals, the elderly, children, and communities whose livelihoods are influenced by climatic patterns, like fisherfolk ( Tran et al., 2013 ; Yenneti et al., 2016 ; Lundgren-Kownacki et al., 2018 ; Pandey and Kumar, 2018 ; Wilk et al., 2018 ). It is likely for India to experience more pronounced impacts of climate change, including disruptions to businesses, damage to infrastructure and homes, complications in mobility, and concerns for public health ( World Bank, 2014 ). Among the most vulnerable are the urban poor, who experience insufficient access to essential resources and services. that could mitigate these risks. About 3.3 to 3.6 billion people reside in environments highly susceptible to climate change impacts. Vulnerability is closely intertwined between humans and ecosystems, with regions facing development constraints being particularly vulnerable to climatic hazards. From 2010 to 2020, regions characterized by high vulnerability saw a 15-fold increase in human fatalities resulting from floods, droughts, and storms when compared to areas with extremely low vulnerability ( IPCC Report, 2022 ).
Resilience refers to the ability of economic, social, and environmental frameworks to withstand hazardous events, disturbances, or trends while maintaining their essential identity, function, and structure. Resilience also includes the capacity to evolve, learn, and adapt ( IPCC, 2014 ). From a vulnerability perspective, incorporating community resources, risk can be defined as: Risk = (Hazard x Vulnerability)/Capacity. In this context, “capacity” refers to the amalgamation of assets and capabilities existing within a community, society, or organization that can reduce the levels of risk or mitigate the consequences of a disaster ( Das et al., 2009 ). Numerous indices evaluate the vulnerability of various communities based on their socio-economic attributes. Indices like the Livelihood Vulnerability Index (LVI), LVI IPCC models, and Climate Vulnerability Index (CVI) are employed to assess climate change vulnerability in different livelihoods within urban communities, as seen in Guwahati city, Assam, India ( Paul et al., 2019 ). Comparative analysis of these vulnerabilities indicated that farmers were the most vulnerable due to their increased sensitivity to health issues and financial setbacks amplified by a restricted ability to adapt. Conversely, doctors exhibited the least vulnerability due to their higher awareness levels and better adaptive capabilities. In addition to that, lowland and floodplain communities face significant vulnerability to climate change-induced extremes. Each year, river floods affect approximately 21 million people worldwide, a figure anticipated to rise to 54 million by 2030 as a result of the combined impacts of climate change and socio-economic expansion ( Luo et al., 2015 ). The Ganga basin, due to its dense population and agricultural dependence, stands out as particularly susceptible to climate change ( Pandey et al., 2022 ). The Brahmaputra basin's climate and geology have induced changes in river channels and led to bank erosion. The immense sediment load deposition in the Ganga-Brahmaputra delta, spanning 1.76 million km 2 , contributes to one of the world's largest fluvial sediment depositions ( Pandey et al., 2022 ). Annual floods deposit about 1,060 Mt of sediment load into the Ganga-Brahmaputra delta and Indian Ocean, constituting a significant alluvial sediment accumulation ( Milliman and Farnsworth, 2013 ). Additionally, inhabitants of the delta regions contend with frequent flooding, sea-level rise, cyclone-induced storm surges, and climate change challenges ( Woodruff et al., 2013 ). Indian states within the flood-prone region of the Ganges-Brahmaputra-Meghna basin experience adverse climate events that elevate vulnerability. Increasing temperatures, shifting rainfall patterns, and intensifying extreme weather events have collectively heightened the landscape risk ( Kishore et al., 2022 ). More frequent and severe floods, driven by intense rainfall and prolonged monsoons, disrupt daily lives, harm infrastructure, and lead to loss of lives. These floods also pose significant challenges to agriculture and food security, as submerged crops result in failed harvests and livelihood losses.
7 Global climate change—Methodologies
Researchers around the globe utilize a variety of climate prediction models based on their climate change variable and subject. Bochenek and Ustrnul (2022) found that artificial neural networks (ANN) and deep learning (DL) were the most dominant algorithms in their analysis, with decision tree methods such as random forests (RF), extreme gradient boosting (XGB), and support vector machines (SVM) also being frequently used. These methods appear to be applicable to both Numerical Weather Prediction (NWP) and climate analysis. Their study also reported that majority of climate change studies are conducted in China, the USA, Australia, India, and Germany.
A 2D Convolutional Neural Network (CNN) has been suggested as a tool for approximating regional precipitation and discharge extremes based on synoptic-scale predictions from general circulation models (GCM) ( Knighton et al., 2019 ). This approach not only allows for the identification of the most reliable fields in estimating extreme precipitation but also facilitates the recognition of important regional and seasonal variations. Machine learning techniques can also enhance the prediction of future intensity-duration-frequency curves, crucial for extreme precipitation and flooding events ( Hu and Ayyub, 2019 ), or assist in estimating trends and seasonal components of rainfall ( Ghaderpour et al., 2021 ).
Projections of emission and greenhouse gas concentration have been made up to 2300 for each Representative Concentration Pathway (RCP) scenario in the Coupled Model Intercomparison Project Phase 5 (CMIP5) and extended to 2500 by Meinshausen et al. (2011) . Similar long-term projections are available up to 2500 for the Shared Socioeconomic Pathways (SSPs) in CMIP6 ( Meinshausen et al., 2020 ). However, no comprehensive climate model results beyond 2300 are currently available. Many studies focusing on time horizons beyond 2100 have relied on Earth System models of reduced complexity or intermediate complexity ( Palmer et al., 2020 ). Lyon et al. (2022) derived preliminary climate projections from the HadCM3 atmosphere-ocean coupled climate model and the TRIFFID dynamic land surface model which highlight the necessity of quantifying the effects of climate change beyond 2100. For instance, global mean temperature continues to raise after 2100 under most emission scenarios, except for the low-emission RCP2.6 scenario. Under the moderate-high RCP6.0 emissions scenario, global mean warming reaches 2.2°C above present-day levels by 2100 and further increases to 3.6°C in 2200 and 4.6°C in 2500.
Choudhury et al. (2023) employed a cellular automata-artificial neural network (CA-ANN) model to forecast the potential scenario of urban heat islands (UHIs) in 2032, indicating an anticipated intensification of land surface temperatures, particularly in rapidly urbanizing regions. Das et al. (2022) utilized a process-based dynamic vegetation modeling (MAPSS-CENTURY: MC) approach to project changes in vegetation life forms under projected climate conditions.
8 Climate change mitigations status and strategies for India
Enabling urban transitions that bring about positive outcomes for promoting mitigation, adaptation, human welfare, the provision of ecosystem services, and decreasing vulnerability within low-income communities necessitates comprehensive, enduring planning that incorporates both physical and natural elements as well as social infrastructure. The following mitigation measures are recommended for developing Asian countries:
i Green and blue infrastructure: Natural and blue infrastructure aids in capturing and storing carbon, and when combined with traditional gray infrastructure, it can decrease energy consumption and minimize risks associated with extreme events like heatwaves, flooding, heavy rainfall, and droughts. Furthermore, it provides supplementary advantages by enhancing health, quality of life, and means of living ( UNEP, 2021 ; IPCC, 2023 ).
ii Climate-smart approaches: Approaches such as climate-resilient agriculture, disaster risk reduction that relies on ecosystems, and the investment in urban blue-green infrastructure effectively tackle adaptation, mitigation, and align with the Sustainable Development Goals all at once ( Jha et al., 2013 ; UNDRR, 2021 ). These options open avenues for climate-resilient development pathways across India.
iii Incorporating climate factors: Decision-making processes at all levels of governance must incorporate climate risks, vulnerabilities, and adaptation strategies. This necessitates a deepened comprehension of climate impacts across sectors and time-space scales, along with enhancements to planning strategies and resource allocation ( IPCC, 2023 ).
iv Enhancing forecasting and decision making: Appropriate prediction of extreme events, improved readiness to risk, and prioritization of individual and collective decision-making are imperative for effective climate action ( UNFCCC, 2008 ; IPCC, 2023 ).
v Transforming climate risks into opportunities: Asian nations can transform climate change risks into opportunities by advancing the quality and sustainability of energy. This includes promoting investments in renewable energy sources, safe-gaurding gas reserves, advancement in water conservation techniques, choosing green infrastructure technologies, and fostering collaborations among various stakeholders ( IPCC, 2023 ).
vi Transitioning from fossil fuels: In light of the current global scenario, a shift from fossil fuel-dependent economies to renewable resources and nature-based solutions is crucial for a sustainable future ( UNDRR, 2021 ).
vii Addressing deforestation and REDD+: The ongoing process of deforestation and the deterioration of forests disrupts their crucial contribution to the worldwide carbon cycle. While REDD+ (Reducing Emissions from Deforestation and Forest Degradation) governance has an important part in mitigating climate change ( Jenkins and Schaap, 2018 ), it requires a holistic approach that navigates through complex institutional landscapes and power dynamics to achieve desired outcomes. However, considering the magnitude of the climate issue and the requisite measures required in other greenhouse gas-emitting sectors, the role of REDD+ has limitations. Implementing REDD+ needs a comprehensive and robust approach ( IPCC, 2023 ).
In conclusion, fostering urban transitions that are beneficial for multiple aspects of sustainability requires holistic planning, integration of various infrastructure types, and a deep understanding of climate impacts and adaptation strategies. The diverse options presented hold promise for addressing climate-related challenges and steering Asian nations toward resilient and sustainable development.
8.1 Nature based solutions as a potential mitigation strategy
The effects of climate change on both nature and people are initially felt within urban settings due to the microcosmic nature of cities and the fact that nearly 50% of world population inhabits urban areas ( Metz et al., 2007 ). The ramifications of climate change on ecosystem functioning and human wellbeing are profound. The escalating frequency of heatwaves, droughts, and flooding further exacerbates socio-economic vulnerabilities. Challenges stemming from urban expansion, such as habitat loss, soil sealing, and increased built-up areas, compound the strain on ecosystem functionality, the delivery of ecosystem services, and human welfare across cities worldwide ( Benedict and McMahon, 2006 ).
Nature-based solutions (NbS) emerge as a promising approach to counter these challenges. NbS capitalize on the inherent benefits of nature to tackle the effects of urbanization and climate change. They encompass interventions that incorporate nature into urban spaces to enhance resilience and wellbeing. One critical avenue of NbS is Urban Green Infrastructure (UGI), which aligns with the principles of NbS. UGI entails the strategic incorporation of green spaces into urban planning across various scales, and it underscores the role of nature in providing a multitude of services to urban populations. This concept stems from urban planning and highlights the pivotal role of green spaces in enhancing ecosystem services. UGI aims to strategically integrate green spaces into urban fabric, recognizing their significance in mitigating adverse climatic effects.
A notable facet of UGI and NbS pertains to the management of urban temperatures. By thoughtfully deploying urban green spaces, cities can significantly alter their microclimates. Urban parks, for instance, have been shown to mitigate temperatures during daytime hours by ~1°C. Notably, larger parks with substantial tree cover exhibit a more pronounced cooling effect ( Bowler et al., 2010 ). Additionally, the nature of surface materials influences the cooling impact. Water bodies, for example, exhibit lower surface temperatures than plantation areas, that are cooler compared to streets and rooftops ( Leuzinger et al., 2010 ). This highlights the potential of water bodies for enhancing cooling effects and suggests concentrating such features in city centers to maximize their impact. Furthermore, the configuration of the urban environment has a critical role to play in the efficacy of temperature regulation efforts. Building structures and layout impact the degree of cooling achieved through green and blue infrastructure. Dense urban areas tend to benefit more from vegetation-based cooling, particularly when considering prevailing wind patterns and times of the day ( Lehmann et al., 2014 ). Individual urban trees also contribute to temperature mitigation by reducing the Urban Heat Island (UHI) effect. The effectiveness of trees in this regard depends on factors like leaf arrangement and canopy shape. Innovative NbS, including green roofs and green walls, further influence urban energy dynamics and thermal moderation. Green walls, for instance, exhibit potential in reducing wall temperatures and mitigating street canyon temperatures, thereby furthering climate adaptation objectives ( Cameron et al., 2014 ).
The choice and supervision of urban plantation amid evolving climate conditions are pivotal for sustained NbS effectiveness. As climate conditions evolve, plant species must be chosen with adaptability in mind. Optimal tree selection involves considerations of cooling efficiency, maintenance requirements, and additional ecosystem services like habitat creation and aesthetic value ( Rahman et al., 2015 ). Design adjustments are necessary to accommodate changing rainfall patterns and increased water demand during droughts. This becomes specifically essential in high-density areas where space is at a premium. Maintaining healthy vegetation cover is essential for preserving ecosystem function. Green roofs, for instance, lower air temperatures by about 1°C and require consistent upkeep to ensure their efficacy ( Speak, 2013 ). Neglecting maintenance compromises the cooling potential of these systems. The albedo effect, influenced by vegetation cover, impacts surface temperatures and underlines the importance of sustained green cover. Vegetation's role in altering building energy balances is another vital aspect. Simulation models highlight that urban greening can significantly reduce energy consumption and lower building temperatures, presenting an alternative to traditional sun-blocking methods ( Yang et al., 2017 ). In urban hydrology, NbS demonstrate their effectiveness by mitigating local flooding and minimizing economic losses during moderate or frequent storm events. However, it's crucial to recognize that these solutions are not very effective in dealing with extensive and devastating occurrences like river floods and intense cloud bursts ( Fletcher et al., 2015 ).
8.2 Indian cities and nature-based solutions
The Blue-Green Master plan of Delhi is a significant initiative aimed at enhancing the city's sustainability and resilience against climate change. This plan focuses on developing a multifunctional blue-green infrastructure that integrates both water and vegetation elements. By combining these elements, the plan seeks to address environmental challenges such as pollution, water scarcity, and inadequate green spaces. It emphasizes the interdependence of water bodies and land, creating a symbiotic relationship that offers various environmental and social benefits. The plan covers strategies for improving water management, green spaces, transportation, energy efficiency, and more, all integrated into a holistic approach for sustainable urban development ( https://dda.org.in/pdf/july13/Final%20MPD%202041%20-%20e%20Gazette_%20English.pdf .).
Rajkot, a city in Gujarat, has developed a comprehensive Climate Change and Environment Action Plan to combat the impact of rising temperatures and climate change. This plan is a collaborative effort between the Climate Change Department, Government of Gujarat, and the Gujarat Ecological Education and Research Foundation. The plan focuses on reducing energy consumption, water conservation, air quality monitoring, waste management, and greenhouse gas reduction. It aims to create a more sustainable and climate-resilient city through a range of strategies, including renewable energy promotion, water conservation, waste reduction, and improved air quality monitoring ( http://www.vasudha-foundation.org/wp-content/uploads/Full-Action-Plan-Rajkot.pdf .). In the case of Bangalore, often referred to as 'Greater Bangalore,' the exponential growth of industries and infrastructure has led to the depletion of green spaces and water bodies, contributing to elevated pollution levels and soaring temperatures. The implementation of NbS have the potential to impart a crucial role in alleviating these challenges. By prioritizing the expansion and preservation of green infrastructure such as parks, urban forests, and gardens, the city can effectively counteract the urban heat island effect and enhance the overall quality of the air ( Nimish et al., 2022 ). This approach not only minimizes the environmental impact of urban expansion but also contributes to the city's long-term resilience against climate change.
Conversely, Nagpur is confronting distinct issues, including water scarcity, flash floods, and air pollution, exacerbated by the ongoing urban expansion. Here, too, NbS offer a promising path toward environmental equilibrium. Innovative strategies like curbside plantation, vertical gardens on buildings, and green roofs mitigate air pollution as well as aid in minimizing the urban heat island effect and enhancing stormwater management during heavy rainfall ( Dhyani et al., 2021 ). Community engagement remains critical for the success of these solutions, with local participation ensuring their sustainability and effectiveness ( Govindarajulu, 2014 ). Preservation of existing green spaces and the restoration of degraded ecosystems are equally important, as they contribute to enhancing biodiversity, maintaining ecological balance, and fostering a more resilient urban environment.
The adoption of sustainable advancements further strengthens the impact of NbS on climate change mitigation. In Bangalore, leveraging technological advancements in construction practices to create green buildings can significantly lower energy consumption while providing a healthier indoor environment ( Smith, 2015 ). Similarly, in Nagpur, the integration of smart city technologies can optimize the allocation of resources for green infrastructure, enhancing the effectiveness of NbS ( Jain et al., 2021 ). The difficulties created by climate change in Bangalore and Nagpur necessitate tailored nature-based solutions that consider the unique characteristics of each city. By embracing green infrastructure expansion, sustainable construction practices, and community engagement, both cities can address their respective environmental issues while paving the way for a more sustainable and resilient future. These endeavors also serve as valuable lessons for other urban areas grappling with similar challenges.
In summation, NbS offer a compelling pathway toward crafting livable, sustainable cities resilient to climate change impacts. The spectrum of vegetation-driven solutions caters to diverse adaptation needs. Strategic planning, informed by modeling and collaboration, is imperative to harness the full potential of NbS. These solutions mitigate the adverse impacts of climate change and represent a paradigm shift toward harmonious coexistence between urban spaces and the natural world.
8.3 Currents gaps and challenges in climate change mitigation for India
Indian cities face significant challenges in leveraging institutional capacities and incentives to harness systemic benefits, acknowledge climate-related synergies and trade-offs ( Revi, 2008 ; Boyd and Ghosh, 2013 ; de Oliveira et al., 2013 ; Sethi and Mohapatra, 2013 ; Chu, 2015 ; Revi et al., 2016 ). After carefully reviewing several papers and reports the following gaps and challenges in climate-change mitigations have been identified:
• The basic environmental challenges faced due to climate change are temperature, altered rainfall patterns, hailstorms, cold waves, etc. that impact human health, livelihood, agriculture and economy.
• The widespread challenges arising from climate change encompass environmental, economic, socio-economic, socio-environmental, natural capital, agricultural vulnerability, water resources, and health issues ( Gupta et al., 2020 ).
• In India, there's a notable absence of a comprehensive understanding of the interaction between climate concerns and developmental objectives at various levels of policy and governance. Short-term, discrete actions undertaken without an assessment of cumulative impacts often succumb to influences of vested interests.
• There exists a gap in adapting holistic strategy that integrates climate change considerations into urbanization planning at any governmental level. Central government initiatives like the Transit Oriented Development Policy, Green Urban Mobility Scheme, Unified Metropolitan Transport Authorities, and notably the Smart Cities Mission aim to achieve integrated urban governance. Urban climate action in cities is influenced by the involvement of higher government levels, which cities often depend on for central schemes and policy direction.
• India's unique socio-economic conditions, diverse geography, and varying climates pose challenges to implementing a uniform policy across the entire nation ( Srivastava, 2021 ).
• The complexity of addressing social and environmental outcomes within REDD+ implementation, reporting, and accountability hampers progress, despite advancements in technology aiding in quantifying forest and carbon changes. Nonetheless, there's still room for improvement in measuring, reporting, and verifying both carbon and non-carbon outcomes. While various global initiatives aim to combat deforestation and forest degradation, they introduce complexities and opportunities for synergies with REDD+.
• Climate Resilient Development (CRD) faces substantial challenges such as fragmented and reactive governance, insufficient evidence for prioritizing actions and sequencing them, as well as financial deficits.
• Modeled trajectories aligned with Nationally Determined Contributions (NDCs) pre-COP26, until 2030, with no post-2030, exhibit higher emissions leading to a global warming of 2.8 [2.1–3.4] °C by 2100. Although many nations declare their aspirations to achieve net zero greenhouse gas (GHG) or net zero carbon dioxide (CO2) emissions by the mid-century, there exists a gap in the scope and specificity of pledges differ, with limited existing policies to fulfill them.
• Numerous obstacles are present in precise forecasting, but they can be overcome through the adoption of advanced technologies, including in situ observation, remote sensing, innovative sensor technologies, citizen science, artificial intelligence, and machine learning tools. Enhanced forecasting capabilities, regional partnerships, improved risk awareness, and leveraging technology contribute to overcoming these challenges.
9 Conclusion
In conclusion, the vulnerability of developing nations in the area of climate change has become an undeniable reality, contrasting starkly with the more developed countries. This susceptibility stems from the intricate interplay of fragile ecosystems, precarious economic structures, and widespread poverty within these developing countries, as elucidated by various scholars ( Panda, 2009 ). The transformations in land use resulting from rapid urbanization, altered river courses, shifting cultivation practices, erosion, and desertification, as outlined by experts ( Chakraborty, 2009 ; Sreenivasulu and Bhaskar, 2010 ) profoundly shifts ecosystems. India, being a rapidly developing nation, has witnessed an alarming warming trend over the decades, leading to unprecedented weather extremes and heightened demand for cooling in the sweltering summers due to both climate change and population growth. It's crucial to underscore that marginalized urban communities, including the impoverished, elderly, children, and those dependent on climatic conditions like fisher folk, will be subjected to impacts of climate change. With larger share of global population dwelling in urban areas ( Metz et al., 2007 ), the path that urban India takes in responding to these challenges holds substantial sway over consumption habits and overall sustainability. In this context, the adoption of nature-based solutions (NbS) emerges as a promising strategy, leveraging nature's innate capacities to counterbalance the impacts of urbanization and climate change. By integrating natural elements into urban landscapes, NbS not only bolster resilience but also promote well-being, offering a ray of hope amidst the encroaching climate crisis.
10 Future directions for climate change studies in India
The future work on climate change in India is a pressing and multifaceted challenge that requires a comprehensive approach to address its far-reaching impacts. India is a geographically diverse country, and future climate research should focus on region-specific impacts. Understanding how climate change affects different parts of India, considering variations in temperature, precipitation, and extreme events, is crucial for developing tailored adaptation strategies. Research should delve into the effects of climate change on agriculture, which remains a lifeline for millions. It's essential to develop climate-resilient farming practices, crop varieties, and water management systems to ensure food security in the face of changing climate patterns. With increasing water stress, studies on sustainable water resource management, including efficient irrigation techniques and groundwater replenishment strategies, are essential. Additionally, India's extensive coastline demands research that prioritizes the development of strategies to mitigate the impact of sea-level rise, including building resilient infrastructure and protecting vulnerable coastal communities. Future research should focus on urban planning, infrastructure development, and efficient energy use to enhance the resilience of cities. Leveraging data, technology, and remote sensing can aid in monitoring climate change and predicting its impacts accurately. Lastly, ensuring that climate policies are aligned with India's development goals and are their effective implementation is a crucial aspect of future work.
Author contributions
SH: Formal analysis, Investigation, Methodology, Resources, Writing – original draft, Writing – review & editing. EH: Formal analysis, Methodology, Writing – original draft. PS: Conceptualization, Investigation, Methodology, Resources, Validation, Visualization, Writing – review & editing. AS: Formal analysis, Investigation, Writing – original draft. PT: Data curation, Investigation, Writing – original draft. SS: Conceptualization, Formal analysis, Investigation, Project administration, Resources, Supervision, Validation, Visualization, Writing – original draft, Writing – review & editing.
The author(s) declare that no financial support was received for the research, authorship, and/or publication of this article.
Conflict of interest
The authors declare that the research was conducted in the absence of any commercial or financial relationships that could be construed as a potential conflict of interest.
Publisher's note
All claims expressed in this article are solely those of the authors and do not necessarily represent those of their affiliated organizations, or those of the publisher, the editors and the reviewers. Any product that may be evaluated in this article, or claim that may be made by its manufacturer, is not guaranteed or endorsed by the publisher.
Abhijith, K., and Kumar, P. (2019). Field investigations for evaluating green infrastructure effects on air quality in open-road conditions. Atmos. Environ. 201, 132–147. doi: 10.1016/j.atmosenv.2018.12.036
Crossref Full Text | Google Scholar
Abhijith, K., Kumar, P., Gallagher, J., McNabola, A., Baldauf, R., Pilla, F., et al. (2017). Air pollution abatement performances of green infrastructure in open road and built-up street canyon environments–a review. Atmosph. Environm . 162, 71–86. doi: 10.1016/j.atmosenv.2017.05.014
Adebayo, T. S., Akadiri, S. S., Riti, J. S., and Tony Odu, A. (2023). Interaction among geopolitical risk, trade openness, economic growth, carbon emissions and its implication on climate change in india. Energy Environ. 34, 1305–1326. doi: 10.1177/0958305X221083236
Ahmad, F., Uddin, M. M., and Goparaju, L. (2018). An evaluation of vegetation health and the socioeconomic dimension of the vulnerability of Jharkhand state of India in climate change scenarios and their likely impact: a geospatial approach. Environm. Socio-Econ. Stud. 6, 39–47. doi: 10.2478/environ-2018-0026
Ahmed, M., Shuai, C., and Ahmed, M. (2022). Influencing factors of carbon emissions and their trends in China and India: a machine learning method. Environ. Sci. Pollut. Res. 29, 48424–48437. doi: 10.1007/s11356-022-18711-3
PubMed Abstract | Crossref Full Text | Google Scholar
Akram, H., Hussain, S., Mazumdar, P., Chua, K. O., Butt, T. E., and Harikrishna, J. A. (2023). Mangrove health: a review of functions, threats, and challenges associated with mangrove management practices. Forests 14, 1698. doi: 10.3390/f14091698
Alexandratos, N., and Bruinsma, J. (2012). World Agriculture: Towards 2030/2050; ESA Working Paper No. 12–03 . Rome, Italy: FAO.
Google Scholar
Amado, M., Poggi, F., and Amado, A. R. (2016). Energy efficient city: a model for urban planning. Sust. Cities Soc. 26, 476–485. doi: 10.1016/j.scs.2016.04.011
Amrit, K., Pandey, R. P., and Mishra, S. K. (2021). “Meteorological drought characteristics in eastern region of India,” in Hydrological Extremes: River Hydraulics and Irrigation Water Management , 111–120.
Barman, S., and Bhattacharjya, R. K. (2015). Change in snow cover area of Brahmaputra river basin and its sensitivity to temperature. Environm. Syst. Res. 4, 1–10. doi: 10.1186/s40068-015-0043-0
Barton, H., and Tsourou, C. (2013). Healthy Urban Planning . London: Routledge. doi: 10.4324/9780203857755
Basha, P. O., and Reddy, M. S. (2022). Importance of standard scientific units for the measurement of quantities and properties in environmental science. Indian J. Adv. Chem. Sci. 10, 163–166. doi: 10.22607/IJACS.2022.1004002
Behera, M. D., Behera, S. K., and Sharma, S. (2019). Recent advances in biodiversity and climate change studies in India. Biodivers. Conserv. 28, 1943–1951. doi: 10.1007/s10531-019-01781-0
Benedict, M. A., and McMahon, E. T. (2006). Green Infrastructure: Linking Landscapes and Communities . Washington, DC: Island Press.
Bibri, S. E., Krogstie, J., and Kärrholm, M. (2020). Compact city planning and development: Emerging practices and strategies for achieving the goals of sustainability. Developments in the built environment 4, 100021. doi: 10.1016/j.dibe.2020.100021
Bochenek, B., and Ustrnul, Z. (2022). Machine learning in weather prediction and climate analyses—applications and perspectives. Atmosphere 13, 180. doi: 10.3390/atmos13020180
Bowler, D. E., Buyung-Ali, L., Knight, T. M., and Pullin, A. S. (2010). Urban greening to cool towns and cities: a systematic review of the empirical evidence. Landsc. Urban Plann. 97, 147–155. doi: 10.1016/j.landurbplan.2010.05.006
Boyd, E., and Ghosh, A. (2013). Innovations for enabling urban climate governance: evidence from Mumbai. Environ. Plann. Govern. Policy 31, 926–945. doi: 10.1068/c12172
Burney, J., and Ramanathan, V. (2014). Recent climate and air pollution impacts on Indian agriculture. Proc. Nat. Acad. Sci. 111, 16319–16324. doi: 10.1073/pnas.1317275111
Cameron, R. W., Taylor, J. E., and Emmett, M. R. (2014). What's ‘cool' in the world of green façades? How plant choice influences the cooling properties of green walls. Build. Environ. 73, 198–207. doi: 10.1016/j.buildenv.2013.12.005
Chakraborty, K. (2009). Vegetation change detection in Barak Basin. Curr. Sci. 96, 1236–1242. Available online at: http://www.jstor.org/stable/24105415
Chaturvedi, R. K., Gopalakrishnan, R., Jayaraman, M., Bala, G., Joshi, N. V., Sukumar, R., et al. (2011). Impact of climate change on Indian forests: a dynamic vegetation modeling approach. Mitig. Adapt. Strateg. Glob. Change 16, 119–142. doi: 10.1007/s11027-010-9257-7
Chaturvedi, R. K., Joshi, J., Jayaraman, M., Bala, G., and Ravindranath, N. H. (2012). Multi-model climate change projections for India under representative concentration pathways. Curr. Sci. 2012, 791–802. Available online at: http://www.jstor.org/stable/24088836
Chauhan, M. S., Sharma, A., Trivedi, A., Kumar, K., Ferguson, D. K., and Rathore, P. S. (2022). Late Quaternary vegetation shifts and climate change in the sub-alpine belt of the Parvati Valley, Himachal Pradesh, India. Quat. Int. 629, 53–64. doi: 10.1016/j.quaint.2020.12.029
Chen, H., Jia, B., and Lau, S. S. Y. (2008). Sustainable urban form for Chinese compact cities: challenges of a rapid urbanized economy. Habitat Int. 32, 28–40. doi: 10.1016/j.habitatint.2007.06.005
Choudhury, U., Singh, S. K., Kumar, A., Meraj, G., Kumar, P., and Kanga, S. (2023). Assessing land use/land cover changes and urban heat island intensification: a case study of Kamrup Metropolitan District, Northeast India (2000–2032). Earth 4, 503–521. doi: 10.3390/earth4030026
Chu, E. (2015). Urban development and climate adaptation: Implications for policymaking and governance in Indian cities. OPPORTUNITIES 6.
Climate India (2022). Available online at: https://www.cseindia.org/climate-india-2022-11463 (accessed September 25, 2023).
Corlett, R. T., and Lafrankie Jr, J. V. (1998). Potential impacts of climate change on tropical Asian forests through an influence on phenology. Clim. Change 39, 439–453. doi: 10.1023/A:1005328124567
Creutzig, F., Fernandez, B., Haberl, H., Khosla, R., Mulugetta, Y., and Seto, K. C. (2016). Beyond technology: demand-side solutions for climate change mitigation. Annu. Rev. Environ. Resour. 41. doi: 10.1146/annurev-environ-110615-085428
D'Amato, G., Calzetta, L., D'Amato, M., and Cazzola, M. (2002). Outdoor air pollution, climatic changes and allergic bronchial asthma. Eur. Respir. J. 20, 763–776. doi: 10.1183/09031936.02.00401402
Das, A., Ghosh, P. K., Choudhury, B. U., Patel, D. P., Munda, G. C., Ngachan, S. V., et al. (2009). “Climate change in North East India: recent facts and events–worry for agricultural management,” in Proceedings of the Workshop on Impact of Climate Change on Agriculture , (32–37).
Das, P., Behera, M. D., Bhaskaran, P. K., and Roy, P. S. (2022). Forest cover resilience to climate change over India using the MC2 dynamic vegetation model. Environ. Monit. Assess. 194, 903. doi: 10.1007/s10661-022-10545-3
de Oliveira, J. A. P., Doll, C. N., Kurniawan, T. A., Geng, Y., Kapshe, M., and Huisingh, D. (2013). Promoting win-win situations in climate change mitigation, local environmental quality and development in Asian cities through co-benefits. J. Clean. Prod. 58, 1–6. doi: 10.1016/j.jclepro.2013.08.011
Dholakia, H. H., and Garg, A. (2018). “Climate change, air pollution and human health in Delhi, India,” in Climate Change and Air Pollution: The Impact on Human Health in Developed and Developing Countries , eds R. Akhtar and C. Palagiano (Cham: Springer International Publishing), 273–288. doi: 10.1007/978-3-319-61346-8_17
Dhyani, S., Majumdar, R., and Santhanam, H. (2021). Scaling-up nature-based solutions for mainstreaming resilience in Indian cities. Ecosyst. Disaster Clim. Resilien. 279–306. doi: 10.1007/978-981-16-4815-1_12
Dhyani, S., Singh, S., Basu, M., Dasgupta, R., and Santhanam, H. (2022). “Blue-green infrastructure for addressing urban resilience and sustainability in the warming world,” in Blue-Green Infrastructure Across Asian Countries: Improving Urban Resilience and Sustainability (Singapore), 1–22. doi: 10.1007/978-981-16-7128-9_1
FAO (2007). “Fire management global assessment 2006,” in FAO Forestry Paper . Rome: FAO, 119.
Fisher, S. (2014). Exploring nascent climate policies in Indian cities: a role for policy mobilities? Int. J. Urban Sustain. Dev. 6, 154–173. doi: 10.1080/19463138.2014.892006
Fletcher, T. D., Shuster, W., Hunt, W. F., Ashley, R., Butler, D., Arthur, S., et al. (2015). SUDS, LID, BMPs, WSUD and more-The evolution and application of terminology surrounding urban drainage. Urban Water J. 12, 525–542. doi: 10.1080/1573062X.2014.916314
Ghaderpour, E., Vujadinovic, T., and Hassan, Q. K. (2021). Application of the least-squares wavelet software in hydrology: athabasca River basin. J. Hydrol.: Reg. Stud. 36, 100847. doi: 10.1016/j.ejrh.2021.100847
Ginsberg, G. L., Foos, B. P., and Firestone, M. P. (2005). Review and analysis of inhalation dosimetry methods for application to children's risk assessment. J. Toxicol. Environ. Health A 68, 573–615. doi: 10.1080/15287390590921793
Goel, A., Saxena, P., Sonwani, S., Rathi, S., Srivastava, A., Bharti, A. K., et al. (2021). Health benefits due to reduction in respirable particulates during COVID-19 lockdown in India. Aerosol Air Qual. Res. 21, 200460. doi: 10.4209/aaqr.200460
Gopalakrishnan, R., Jayaraman, M., Bala, G., and Ravindranath, N. H. (2011). Climate change and Indian forests. Curr. Sci. 348–355.
Govindarajulu, D. (2014). Urban green space planning for climate adaptation in Indian cities. Urban Clim. 10, 35–41. doi: 10.1016/j.uclim.2014.09.006
Green, E., Short, S., Stutt, E., and Harrison, P. (2000). Protecting environmental quality and human health: strategies for harmonisation. Sci. Total Environ. 256, 205–213. doi: 10.1016/S0048-9697(00)00493-9
Guntukula, R. (2020). Assessing the impact of climate change on Indian agriculture: evidence from major crop yields. J. Public Affairs 20, e2040. doi: 10.1002/pa.2040
Gupta, A. K., Negi, M., Nandy, S., Kumar, M., Singh, V., Valente, D., et al. (2020). Mapping socio-environmental vulnerability to climate change in different altitude zones in the Indian Himalayas. Ecol. Indic. 109, 105787. doi: 10.1016/j.ecolind.2019.105787
Gupta, R., Somanathan, E., and Dey, S. (2017). Global warming and local air pollution have reduced wheat yields in India. Clim. Change 140, 593–604. doi: 10.1007/s10584-016-1878-8
Gurjar, B. R., Ohara, T., Khare, M., Kulshrestha, P., Tyagi, V., and Nagpure, A. S. (2018). “South Asian perspective: a case of urban air pollution and potential for climate co-benefits in India,” in Mainstreaming Climate Co-benefits in Indian Cities: Post-habitat III Innovations and Reforms , 77–98. doi: 10.1007/978-981-10-5816-5_3
Haibach, H., and Schneider, K. (2013). “The politics of climate change: review and future challenges,” in Climate Change: International Law and Global Governance . Baden-Baden: Nomos VerlagsgesellschaftmbHand Co. KG, 357–374.
Harrison, R. M., and Yin, J. (2000). Particulate matter in the atmosphere: which particle properties are important for its effects on health? Sci . Total Environ. 249, 85–101. doi: 10.1016/S0048-9697(99)00513-6
Haughan, A. E., Pettorelli, N., Potts, S. G., and Senapathi, D. (2022). Determining the role of climate change in India's past forest loss. Glob. Chang. Biol. 28, 3883–3901. doi: 10.1111/gcb.16161
Heal, M. R., Kumar, P., and Harrison, R. M. (2012). Particles, air quality, policy and health. Chem. Soc. Rev. 41, 6606–6630. doi: 10.1039/c2cs35076a
Hoegh-Guldberg, O., Jacob, D., Taylor, M., Bindi, M., Brown, S., Camilloni, I., et al. (2018). “Impacts of 1.5°C global warming on natural and human systems,” in Global Warming of 1.5°C. An IPCC Special Report on the Impacts of Global Warming of 1.5°C Above Pre-Industrial Levels and Related Global Greenhouse Gas Emission Pathways, in the Context of Strengthening the Global Response to the Threat of Climate Change, Sustainable Development, and Efforts to Eradicate Poverty , eds. V. Masson-Delmotte, P. Zhai, H.-O. Pörtner, D. Roberts, J. Skea, P. R. Shukla, et al. Cambridge and New York: Cambridge University Press, 175–312.
Hu, H., and Ayyub, B. M. (2019). Machine learning for projecting extreme precipitation intensity for short durations in a changing climate. Geosciences 9, 209. doi: 10.3390/geosciences9050209
Hussain, S., and Hoque, R. R. (2022a). “Ecological and natural-based solutions as green growth strategies for disaster and emergency management of air pollution extremes,” in Extremes in Atmospheric Processes and Phenomenon: Assessment, Impacts and Mitigation . Singapore: Springer Nature Singapore, 369–395.
Hussain, S., and Hoque, R. R. (2022b). Biomonitoring of metallic air pollutants in unique habitations of the Brahmaputra Valley using moss species—Atrichumangustatum: Spatiotemporal deposition patterns and sources. Environ. Sci. Pollut. Res. Int. 29, 10617–10634. doi: 10.1007/s11356-021-16153-x
IMD Annual Report (2021). India Meteorological Department, New Delhi [Information Science & Knowledge Resource Development Division (IS&KRDD), (Formerly Publication Section)] oES/IMD/Annual Report -2022/(01)2023/02.
International Council of Local Environmental Initiatives-South Asia (2014). CLEI South Asia. New Delhi. 1–31 . Available online at: https://southasia.iclei.org/wp-content/uploads/2021/08/Annual-Report-2013-2014.pdf
IPCC (2014). AR5 Synthesis Report: Climate Change 2014. Avaialble online at: https://www.ipcc.ch/report/ar5/syr/
IPCC (2023). AR6 Synthesis Report: Climate Change 2023 . Available online at: https://www.ipcc.ch/report/sixth-assessment-report-cycle/
IPCC Report (2022). Climate Change 2022: Impacts, Adaptation and Vulnerability . Available online at: https://www.ipcc.ch/report/ar6/wg2/
IUFRO Report (2020). Available online at: https://www.iufro.org/fileadmin/material/publications/annual-reports/ar22.pdf (accessed August 07, 2023).
Jain, M., Saxena, P., Sharma, S., and Sonwani, S. (2021). Investigation of forest fire activity changes over the central India domain using satellite observations during 2001–2020. GeoHealth 5, e2021GH000528. doi: 10.1029/2021GH000528
Janes, T., McGrath, F., Macadam, I., and Jones, R. (2019). High-resolution climate projections for South Asia to inform climate impacts and adaptation studies in the Ganges-Brahmaputra-Meghna and Mahanadi deltas. Sci. Total Environ. 650, 1499–1520. doi: 10.1016/j.scitotenv.2018.08.376
Jenkins, M., and Schaap, B. (2018). Forest Ecosystem Services. Background Analytical Study , 1. United Nations Form on Forests.
Jha, A. K., Miner, T. W., and Stanton-Geddes, Z. (2013). Building Urban Resilience: Principles, Tools, and Practice . Washington, DC: World Bank Publications. doi: 10.1596/978-0-8213-8865-5
Kam, J., Knutson, T. R., Zeng, F., and Wittenberg, A. T. (2016). Multimodel assessment of anthropogenic influence on record global and regional warmth during 2015. Bull. Assoc. Eng. Geol. 97, S4–S8. doi: 10.1175/BAMS-D-16-0138.1
Karak, T., Bhagat, R. M., and Bhattacharyya, P. (2012). Municipal solid waste generation, composition, and management: the world scenario. Critic. Rev. Environ. Sci. Technol. 42, 1509–1630. doi: 10.1080/10643389.2011.569871
Khosla, R., and Bhardwaj, A. (2019). Urbanization in the time of climate change: examining the response of Indian cities. Wiley Interdisc. Rev. 10:e560. doi: 10.1002/wcc.560
Kinney, P. L. (2018). Interactions of climate change, air pollution, and human health. Curr. Environm. Health Rep. 5, 179–186. doi: 10.1007/s40572-018-0188-x
Kirschbaum, M. U. F., Cannell, M. G. R., Cruz, R. V. O., Galinski, W., and Cramer, W. P. (1996). “Climate change impacts on forests,” in Climate Change 1995. Impacts, Adaptation and Mitigation of Climate Change: Scietific-Technical Analyses , eds. R. T. Watson, M. C. Zinyowera, R. H. Moss, and D. J. Dokken. Cambridge: Cambridge University Press.
Kishore, P., Basha, G., Ratnam, M. V., AghaKouchak, A., Velicogna, I., and Rajeevan, M. (2022). Precipitation variability over India during the 20th and 21st centuries: investigating natural and anthropogenic drivers. Clim. Change 172, 37. doi: 10.1007/s10584-021-03068-2
Kitoh, A. (2017). The Asian monsoon and its future change in climatemodels: a review. J. Meteorol. Soc. Japan. Ser. II 95, 7–33. doi: 10.2151/jmsj.2017-002
Klingenfeld, D., and Schellnhuber, H. J. (2012). Climate Change as a Global Challenge–and its Implications for Knowledge Generation and Dissemination .
Knighton, J., Pleiss, G., Carter, E., Lyon, S., Walter, M. T., and Steinschneider, S. (2019). Potential predictability of regional precipitation and discharge extremes using synoptic-scale climate information via machine learning: An evaluation for the eastern continental United States. J. Hydrometeorol. 20, 883–900. doi: 10.1175/JHM-D-18-0196.1
Krishnan, R., Sanjay, J., Gnanaseelan, C., Mujumdar, M., Kulkarni, A., and Chakraborty, S. (2020). Assessment of Climate Change Over the Indian Region: A Report of the Ministry of earth Sciences (MOES), Government of India . Cham: Springer Nature, p. 226.
Kumar, P., Druckman, A., Gallagher, J., Gatersleben, B., Allison, S., Eisenman, T. S., et al. (2019). The nexus between air pollution, green infrastructure and human health. Environm. Int. 133, 105181. doi: 10.1016/j.envint.2019.105181
Kumar, P., Morawska, L., Martani, C., Biskos, G., Neophytou, M., Di Sabatino, S., et al. (2015). The rise of low-cost sensing for managing air pollution in cities. Environ. Int. 75, 199–205. doi: 10.1016/j.envint.2014.11.019
Lehmann, J., Coumou, D., Frieler, K., Eliseev, A. V., and Levermann, A. (2014). Future changes in extratropical storm tracks and baroclinicity under climate change. Environ. Res. Lett. 9:84002. doi: 10.1088/1748-9326/9/8/084002
Lele, S., Srinivasan, V., Thomas, B. K., and Jamwal, P. (2018). Adapting to climate change in rapidly urbanizing river basins: insights from a multiple-concerns, multiple-stressors, and multi-level approach. Water Int. 43, 281–304. doi: 10.1080/02508060.2017.1416442
Leuzinger, S., Vogt, R., and Körner, C. (2010). Tree surface temperature in an urban environment. Agric. Forest Meteorol. 150, 56–62. doi: 10.1016/j.agrformet.2009.08.006
Liang, Z., and Qamruzzaman, M. (2022). An asymmetric investigation of the nexus between economic policy uncertainty, knowledge spillover, climate change, and green economy: Evidence from BRIC nations. Front. Environm. Sci. 9, 682. doi: 10.3389/fenvs.2021.807424
Liu, X., Huang, Y., Xu, X., Li, X., Li, X., Ciais, P., et al. (2020). High-spatiotemporal-resolution mapping of global urban change from 1985 to 2015. Nat. Sustain. 3, 564–570. doi: 10.1038/s41893-020-0521-x
Loukas, A., Garrote, L., and Vasiliades, L. (2021). Hydrological and hydro-meteorological extremes and related risk and uncertainty. Water 13, 377. doi: 10.3390/w13030377
Lundgren-Kownacki, K., Kjellberg, S. M., Gooch, P., Dabaieh, M., Anandh, L., and Venugopal, V. (2018). Climate change-induced heat risks for migrant populations working at brick kilns in India: a transdisciplinary approach. Int. J. Biometeorol. 62, 347–358. doi: 10.1007/s00484-017-1476-0
Luo, T., Maddocks, A., Iceland, C., Ward, P., and Winsemius, H. (2015). World's 15 Countries With the Most People Exposed to River Floods .
Lutz, A. F., Immerzeel, W. W., Shrestha, A. B., and Bierkens, M. F. P. (2014). Consistent increase in High Asia's runoff due to increasing glaciermelt and precipitation. Nat. Clim. Chang. 4, 587–592 doi: 10.1038/nclimate2237
Lyon, C., Saupe, E. E., Smith, C. J., Hill, D. J., Beckerman, A. P., Stringer, L. C., et al. (2022). Climate change research and action must look beyond 2100. Glob. Chang. Biol. 28, 349–361. doi: 10.1111/gcb.15871
Maji, S., and Sonwani, S. (2022). “Nature of Sand and Dust Storm in South Asian Region: Extremities and Environmental Impacts,” in Extremes in Atmospheric Processes and Phenomenon: Assessment, Impacts and Mitigation . Singapore: Springer Nature Singapore, 113–139.
Malhi, G. S., Kaur, M., and Kaushik, P. (2021). Impact of climate change on agriculture and its mitigation strategies: a review. Sustainability 13, 1318. doi: 10.3390/su13031318
Manish, K., Telwala, Y., Nautiyal, D. C., and Pandit, M. K. (2016). Modelling the impacts of future climate change on plant communities in the Himalaya: a case study from Eastern Himalaya, India. Model. Earth Syst. Environm. 2, 1–12. doi: 10.1007/s40808-016-0163-1
Marchin, R. M., Backes, D., Ossola, A., Leishman, M. R., Tjoelker, M. G., and Ellsworth, D. S. (2022). Extreme heat increases stomatal conductance and drought-induced mortality risk in vulnerable plant species. Glob. Chang. Biol. 28, 1133–1146. doi: 10.1111/gcb.15976
Mathiarasan, S., and Hüls, A. (2021). Impact of environmental injustice on children's health—interaction between air pollution and socioeconomic status. Int. J. Environ. Res. Public Health 18, 795. doi: 10.3390/ijerph18020795
Matyssek, R., Wieser, G., Calfapietra, C., De Vries, W., Dizengremel, P., and Ernst, D. etale (2012). Forests under climate change and air pollution: gaps in understanding and future directions for research. Environ. Pollut. 160, 57–65. doi: 10.1016/j.envpol.2011.07.007
Meinshausen, M., Nicholls, Z. R. J., Lewis, J., Gidden, M. J., Vogel, E., Freund, M., et al. (2020). The shared socio-economic pathway (SSP) greenhouse gas concentrations and their extensions to 2500. Geosci. Model Dev. 13, 3571–3605. doi: 10.5194/gmd-13-3571-2020
Meinshausen, M., Smith, S. J., Calvin, K., Daniel, J. S., Kainuma, M. L. T., Lamarque, J.-F., et al. (2011). The RCP greenhouse gas concentrations and their extensions from 1765 to 2300. Clim. Change 109, 213–241. doi: 10.1007/s10584-011-0156-z
Metz, B., Davidson, O., Bosch, P., Dave, R., and Meyer, L. (2007). Mitigation of Climate Change . Contribution of Working Group II to the Fourth Assessment Report of the Intergovernmental Panel on Climate Change (IPCC).
Milliman, J. D., and Farnsworth, K. L. (2013). River Discharge to the Coastal Ocean: A Global Synthesis. Cambridge: Cambridge University Press.
Mukherjee, A., Bhanja, S. N., and Wada, Y. (2018). Groundwater depletion causing reduction of baseflow triggering Ganges river summer drying. Sci. Rep. 8, 12049. doi: 10.1038/s41598-018-30246-7
Muñoz-Pizza, D. M., Villada-Canela, M., Rivera-Castañeda, P., Osornio-Vargas, Á., Martínez-Cruz, A. L., and Texcalac-Sangrador, J. L. (2022). Barriers and opportunities to incorporate scientific evidence into air quality management in Mexico: a stakeholders' perspective. Environ. Sci. Policy 129, 87–95. doi: 10.1016/j.envsci.2021.12.022
Nandi, S., and Swain, S. (2022). Analysis of heatwave characteristics under climate change over three highly populated cities of South India: a CMIP6-based assessment. Environ. Sci. Pollut. Res. 2022, 1–13. doi: 10.1007/s11356-022-22398-x
Nanditha, J. S., van der Wiel, K., Bhatia, U., Stone, D., Selton, F., and Mishra, V. (2020). A seven-fold rise in the probability of exceeding the observed hottest summer in India in a 2 C warmer world. Environm. Res. Lett. 15, 044028. doi: 10.1088/1748-9326/ab7555
National Oceanic and Atmospheric Administration (NOAA) (2021). Historical El Nino/La Nina episodes (1950-present) . Available online at: https://origin.cpc.ncep.noaa.gov/products/analysis_monitoring/ensostuff/ONI_v5.php (accessed September 10, 2023).
Nimish, G., Bharath, H. A., and Ramachandra, T. V. (2022). “Visualization of landuse change pattern and its impact on Urban Heat Islands,” in Global Urban Heat Island Mitigation (Elsevier), 301–322. doi: 10.1016/B978-0-323-85539-6.00001-9
Nixon, J. D., Dey, P. K., Ghosh, S. K., and Davies, P. A. (2013). Evaluation of options for energy recovery from municipal solid waste in India using the hierarchical analytical network process. Energy 59, 215–223. doi: 10.1016/j.energy.2013.06.052
Nurdiati, S., Bukhari, F., Julianto, M. T., Sopaheluwakan, A., Aprilia, M., Fajar, I., et al. (2022). The impact of El Niño southern oscillation and Indian Ocean Dipole on the burned area in Indonesia. Terrestrial, Atmosph. Oceanic Sci. 33, 16. doi: 10.1007/s44195-022-00016-0
OECD (2023). Air and GHG Emissions (Indicator) .
Pai, D. S., and Nair, S. (2022). Impact of El-Niño-Southern Oscillation (ENSO) on extreme temperature events over India. MAUSAM 73, 597–606. doi: 10.54302/mausam.v73i3.5932
Palmer, M. D., Gregory, J. M., Bagge, M., Calvert, D., Hagedoorn, J. M., Howard, T., et al. (2020). Exploring the drivers of global and local sea-level change over the 21st century and beyond. Earth's Future 8, 9. doi: 10.1029/2019EF001413
Panda, A. (2009). Assessing vulnerability to climate change in India. Econ. Polit. Wkly. 44, 105–107. Available online at: http://www.jstor.org/stable/40279163
Panda, D. K., AghaKouchak, A., and Ambast, S. K. (2017). Increasing heat waves and warm spells in India, observed from a multiaspect framework. J. Geophys. Res. 122, 3837–3858. doi: 10.1002/2016JD026292
Pandey, A. C., Kaushik, K., and Parida, B. R. (2022). Google Earth Engine for large-scale flood mapping using SAR data and impact assessment on agriculture and population of Ganga-Brahmaputra basin. Sustainability 14, 4210. doi: 10.3390/su14074210
Pandey, U. C., and Kumar, C. (2018). Emerging paradigms of capacity building in the context of climate change. Clim. Liter. Innov. Clim. Change Educ. 193–214. doi: 10.1007/978-3-319-70199-8_11
Parikh, S., and Bhavsar, D. (2023). A review: urban forest and climate change. Int. Assoc. Biol. Comput. Dig. 2, 287–294. doi: 10.56588/iabcd.v2i1.176
Patel, L., Conlon, K. C., Sorensen, C., McEachin, S., Nadeau, K., Kakkad, K., et al. (2022). Climate change and extreme heat events: how health systems should prepare. NEJM Catalyst Innovat. Care Deliv . 3, CAT-21. doi: 10.1056/CAT.21.0454
Pathak, H. (2023). Impact, adaptation, and mitigation of climate change in Indian agriculture. Environ. Monit. Assess. 195, 52. doi: 10.1007/s10661-022-10537-3
Paul, A., Deka, J., Gujre, N., Rangan, L., and Mitra, S. (2019). Does nature of livelihood regulate the urban community's vulnerability to climate change? Guwahati city, a case study from North East India. J. Environ. Manage. 251, 109591. doi: 10.1016/j.jenvman.2019.109591
Perera, F. (2018). Pollution from fossil-fuel combustion is the leading environmental threat to global pediatric health and equity: solutions exist. Int. J. Environ. Res. Public Health 15, 16. doi: 10.3390/ijerph15010016
Perera, F. P. (2017). Multiple threats to child health from fossil fuel combustion: impacts of air pollution and climate change. Environ. Health Perspect. 125, 141. doi: 10.1289/EHP299
Rahman, M. A., Armson, D., and Ennos, A. R. (2015). A comparison of the growth and cooling effectiveness of five commonly planted urban tree species. Urban Ecosyst. 18, 371–389.
Rajasekar, U., Chakraborty, S., and Bhat, G. (2018). Climate resilient smart cities: opportunities for innovative solutions in India. Clim. Change Cities 203–227. doi: 10.1007/978-3-319-65003-6_11
Ramanathan, V., and Carmichael, G. (2008). Global and regional climate changes due to black carbon. Nat. Geosci. 1, 221–227. doi: 10.1038/ngeo156
Ranjan, A. K., and Gorai, A. K. (2022). Evaluating phenological trends of different vegetation types in response to climate change over the Rajmahal Hills in India during 2001-2019. Remote Sensing Lett. 13, 898–911. doi: 10.1080/2150704X.2022.2106455
Ranjan, P., and Narain, V. (2012). “Urbanization, climate change and water security: a study of vulnerability and adaptation in Sultanpur and Jhanjhrola Khera in peri-urban Gurgaon, India” in Peri-Urban Water Security Discussion Paper Series, Paper 3.
Ravindra, K., Rattan, P., Mor, S., and Aggarwal, A. N. (2019). Generalized additive models: Building evidence of air pollution, climate change and human health. Environ. Int. 132, 104987. doi: 10.1016/j.envint.2019.104987
Ren, G., Zhan, Y., Ren, Y., Wen, K., Zhang, Y., Sun, X., et al. (2023). Observed changes in temperature and precipitation over Asia, 1901-2020. Climate Res. 90, 31–43. doi: 10.3354/cr01713
Revi, A. (2008). Climate change risk: an adaptation and mitigation agenda for Indian cities. Environ. Urbaniz. 20, 207–229. doi: 10.1177/0956247808089157
Revi, A., Gajjar, S. P., Basu, R., Jain, G., and Bazaz, A. B. (2016). Cities on a Finite Planet. Bangalore: Routledge.
Roy, J., Chakravarty, D., Dasgupta, S., Chakraborty, D., Pal, S., and Ghosh, D. (2018). Where is the hope? Blending modern urban lifestyle with cultural practices in India. Curr. Opin. Environ. Sustain. 31, 96–103. doi: 10.1016/j.cosust.2018.01.010
Roy, P., Pal, S. C., Chakrabortty, R., Chowdhuri, I., Saha, A., and Shit, M. (2022). Climate change and groundwater overdraft impacts on agricultural drought in India: vulnerability assessment, food security measures and policy recommendation. Sci. Total Environ. 849, 157850. doi: 10.1016/j.scitotenv.2022.157850
Sahu, L. K., and Saxena, P. (2015). High time and mass resolved PTR-TOF-MS measurements of VOCs at an urban site of India during winter: role of anthropogenic, biomass burning, biogenic and photochemical sources. Atmosph. Res. 164, 84–94. doi: 10.1016/j.atmosres.2015.04.021
Saini, S., Aggarwal, S., and Punhani, G. (2015). “Urban poor women and climate change in India: Enhancing adaptive capacity through communication for development,” in Climate change in the Asia-Pacific Region , ed W. Leal Filho (Cham: Springer), 67–88.
Salunke, A. Y. (2022). Unravelling the Genotoxic Potential of Agrochemicals on Fish Cell Line (Doctoral dissertation). Vadodara: Maharaja Sayajirao University of Baroda (India).
Sannigrahi, S., Pilla, F., Basu, B., Basu, A. S., Sarkar, K., Chakraborti, S., et al. (2020). Examining the effects of forest fire on terrestrial carbon emission and ecosystem production in India using remote sensing approaches. Sci. Total Environ. 725, 138331. doi: 10.1016/j.scitotenv.2020.138331
Satendra, A., and Kaushik, A. D. (2014), Forest Fire Disaster Management . New Delhi: National Institute of Disaster Management, Ministry of Home Affairs, Government of India.
Sathaye, J., Shukla, P. R., and Ravindranath, N. H. (2006). Climate change, sustainable development and India: global and national concerns. Curr. Sci. 90, 314–325. Available online at: http://www.jstor.org/stable/24091865
Saurabh Sonwani, S. S., and Vandana Maurya, V. M. (2019). “Impact of air pollution on the environment and economy,” in Air Pollution: Sources, Impacts and Controls (Wallingford, UK: CAB International), 113–134. doi: 10.1079/9781786393890.0113
Saxena, P., Shukla, A., and Gupta, A. K. (2022). Extremes in Atmospheric Processes and Phenomenon: Assessment, Impacts and Mitigation. Cham: Springer.
Saxena, P., and Sonwani, S. (2019a). “Secondary criteria air pollutants: environmental health effects,” in Criteria Air Pollutants and Their Impact on Environmental Health , 83–126.
Saxena, P., and Sonwani, S. (2019b). Criteria Air Pollutants and Their Impact on Environmental Health. Singapore: Springer Singapore.
Saxena, P., and Sonwani, S. (2020). Remediation of ozone pollution by ornamental plants in indoor environment. Global J. Environm. Sci. Manage. 6, 497–508. Available online at: https://doi.org/10.22034/gjesm.2020.04.06
Saxena, P., and Srivastava, A. (2020). Air Pollution and Environmental Health . Singapore: Springer, 7–253.
Semba, R. D., Askari, S., Gibson, S., Bloem, M. W., and Kraemer, K. (2022). The potential impact of climate change on the micronutrient-rich food supply. Adv. Nutr. 13, 80–100. doi: 10.1093/advances/nmab104
Seppälä, R., Buck, A., and Katila, P. (2009). Adaptation of Forests and People to Climate change: A Global Assessment Report . Helsinki: IUFRO World Series.
Sethi, M., and Mohapatra, S. (2013). “Governance framework to mitigate climate change: challenges in urbanising India,” in Governance Approaches to Mitigation of and Adaptation to Climate Change in Asia (London: Palgrave Macmillan UK), 200–230.
Sharma, A. (2016). Life Cycle Economic and Environmental Impact Assessment of Alternative Transport Fuels and Power-Train Technologies .
Sharma, A., and Kumar, P. (2018). A review of factors surrounding the air pollution exposure to in-pram babies and mitigation strategies. Environ. Int. 120, 262–278. doi: 10.1016/j.envint.2018.07.038
Sharma, A., and Kumar, P. (2020). Quantification of air pollution exposure to in-pram babies and mitigation strategies. Environ. Int. 139, 105671. doi: 10.1016/j.envint.2020.105671
Shrestha, S. (2019). Effects of climate change in agricultural insect pest. Acta Sci. Agric 3, 74–80. doi: 10.31080/ASAG.2019.03.0727
Singh, C., Madhavan, M., Arvind, J., and Bazaz, A. (2021). Climate change adaptation in Indian cities: A review of existing actions and spaces for triple wins. Urban Climate 36, 100783. doi: 10.1016/j.uclim.2021.100783
Singh, D., Horton, D. E., Tsiang, M., Haugen, M., Ashfaq, M., Mei, R., et al. (2014). Severe precipitation in Northern India in June 2013: causes, historical context, and changes in probability. Bull. Am. Meteorol. Soc. 95, S58–S61.
Singh, P., Sarkar Chaudhuri, A., Verma, P., Singh, V. K., and Meena, S. R. (2022). Earth observation data sets in monitoring of urbanization and urban heat island of Delhi, India. Geomat. Nat. Hazards Risk 13, 1762–1779. doi: 10.1080/19475705.2022.2097452
Singh, P., and Yadav, D. (2021). “Link between air pollution and global climate change,” in Global Climate Change (Elsevier), 79–108. doi: 10.1016/B978-0-12-822928-6.00009-5
Singh, R. K., Datta, M., Nema, A. K., and Pérez, I. V. (2013). Evaluating groundwater contamination hazard rating of municipal solid waste landfills in India and Europe using a new system. J. Hazardous Toxic Radio. Waste 17, 62–73. doi: 10.1061/(ASCE)HZ.2153-5515.0000145
Smith, R. M. (2015). “Green” building in India: a comparative and spatial analysis of the LEED-India and GRIHA rating systems. Asian Geograph. 32, 73–84. doi: 10.1080/10225706.2015.1020065
Sonwani, S. (2016). “Source apportionment of polycyclic aromatic hydrocarbons in urban atmosphere of South Delhi, India,” in 2nd International Conference on Atmospheric Dust-DUST2016, Pro Science Conference Proceedings of Scientific Events (Bari), 111–116.
Sonwani, S., Hussain, S., and Saxena, P. (2022b). Air pollution and climate change impact on forest ecosystems in Asian region–a review. Ecosyst. Health Sustain. 8, 2090448. doi: 10.1080/20964129.2022.2090448
Sonwani, S., and Kulshrestha, U. C. (2019). PM 10 carbonaceous aerosols and their real-time wet scavenging during monsoon and non-monsoon seasons at Delhi, India. J. Atmos. Chem. 76, 171–200. doi: 10.1007/s10874-019-09396-z
Sonwani, S., Madaan, S., Arora, J., Suryanarayan, S., Rangra, D., Mongia, N., et al. (2021a). Inhalation exposure to atmospheric nanoparticles and its associated impacts on human health: a review. Front. Sust. Cities 3, 690444. doi: 10.3389/frsc.2021.690444
Sonwani, S., and Saxena, P. (2022). Greenhouse Gases: Sources, Sinks and Mitigation . Singapore: Springer.
Sonwani, S., Saxena, P., and Khillare, P. S. (2022a). Profile of atmospheric particulate PAHs near busy roadway in tropical megacity, India. Inhal. Toxicol. 34, 39–50. doi: 10.1080/08958378.2022.2030442
Sonwani, S., Saxena, P., and Shukla, A. (2021b). Carbonaceous aerosol characterization and their relationship with meteorological parameters during summer monsoon and winter monsoon at an industrial region in Delhi, India. Earth Space Sci . 8, e2020EA001303. doi: 10.1029/2020EA001303
Sonwani, S., and Shukla, A. (2022). Airborne Particulate Matter: Source, Chemistry and Health . Springer Nature. doi: 10.1007/978-981-16-5387-2
Sonwani, S., Yadav, A., and Saxena, P. (2021c). Atmospheric brown carbon: a global emerging concern for climate and environmental health. Manage. Contami. Emerg. Concern (CEC) Environm. 1, 225–247. doi: 10.1016/B978-0-12-822263-8.00008-7
Speak, A. (2013). Quantification of the Environmental Impacts of Urban Green Roofs. The University of Manchester.
Sreenivasulu, V., and Bhaskar, P. U. (2010). Change detection in landuse and landcover using remote sensing and GIS techniques. Int. J. Eng. Sci Technol. 2, 7758−7762.
Srivastava, S. K. (2021). “New challenges on natural resources and their impact on climate change in the indian context,” in India: Climate Change Impacts, Mitigation and Adaptation in Developing Countries . Cham: Springer International Publishing, 1–15.
Stevanovic, M., Popp, A., Campen, H. L., Dietrich, J. P., Muller, C., Bonsch, M., et al. (2016). The impact of high-end climate change on agricultural welfare. Sci. Adv . 2. E1501452. doi: 10.1126/sciadv.1501452
Subramanian, A., Nagarajan, A. M., Vinod, S., Chakraborty, S., Sivagami, K., Theodore, T., et al. (2023). Long-term impacts of climate change on coastal and transitional eco-systems in India: an overview of its current status, future projections, solutions, and policies. RSC Adv. 13, 12204–12228. doi: 10.1039/D2RA07448F
Taraporevala, P. (2018). Demystifying the Indian Smart City. New Delhi: Centre for Policy Research.
Tewar, M., Aziz, Z., Cook, M., Goldar, A., Ray, I., Ray, S., et al. (2015). Reimagining India's Urban Future: A Framework For Securing High-Growth, Low-Carbon, Climate-Resilient Urban Development In India.
Tiseo, I. (2023a). Distribution of GHG Emissions in India 2020, by Sector, Energy and Emissions, Statista .
Tiseo, I. (2023b). Per capita Carbon Dioxide (CO 2 ) Emissions From Fossil Fuels in India from 1970 to 2022, Energy and Emissions, Statista .
Tiseo, I. (2023c). Carbon Dioxide (CO 2 ) Emissions From Fossil Fuel and Industrial Purposes in India from 1970 to 2022, Energy and Emissions, Statista .
Tran, K. V., Azhar, G. S., Nair, R., Knowlton, K., Jaiswal, A., Sheffield, P., et al. (2013). A cross-sectional, randomized cluster sample survey of household vulnerability to extreme heat among slum dwellers in Ahmedabad, India. Int. J. Environ. Res. Public Health 10, 2515–2543. doi: 10.3390/ijerph10062515
UN (2022). Available online at: https://www.un.org/en/observances/seagrass-day (accessed September 25, 2023).
UNDP (2014). Available online at: https://www.undp.org/publications/undp-annual-report-2014
UNDRR (2021). Integrating Disaster Risk Reduction and Climate Change Adaptation in the UN Sustainable Development Cooperation Framework . United Nations Office for Disaster Risk Reduction.
UNEP (2019). Emissions Gap Report . Nairobi, Kenya: United Nations Environment Programme.
UNEP (2021). Available online at: https://www.unep.org/annualreport/2021/index.php
UNFCCC (2008). Report on the Workshop on Climate Modelling, Scenarios and Downscaling Under the NWP on Impacts, Vulnerability and Adaptation to Climate Change . Bonn: FCCC/SBSTA/2008/9.UNFCCC.
United Nations Environment Programme (2009). Recent Trends in Melting Glaciers, Tropospheric Temperatures Over the Himalayas and Summer Monsoon Rainfall over India . Available online at: https://wedocs.unep.org/20.500.11822/8536
United Nations Environment Programme (2021). A Practical Guide to Climate-resilient Buildings and Communities . Nairobi: UNEP.
Upgupta, S., Sharma, J., Jayaraman, M., Kumar, V., and Ravindranath, N. H. (2015). Climate change impact and vulnerability assessment of forests in the Indian Western Himalayan region: A case study of Himachal Pradesh, India. Climate Risk Manage. 10, 63–76. doi: 10.1016/j.crm.2015.08.002
Ürge-Vorsatz, D., Rosenzweig, C., Dawson, R. J., Sanchez Rodriguez, R., Bai, X., Barau, A. S., et al. (2018). Locking in positive climate responses in cities. Nat. Clim. Change 8, 174–177.
van Oldenborgh, G. J., Otto, F. E. L., Haustein, K., and Achuta, R. K. (2016). The heavy precipitation event of December 2015 in Chennai, India. Bull. Am. Meteorol. Soc. 97, S87–S91. doi: 10.1175/BAMS-D-16-0129.1
Venter, Z. S., Aunan, K., Chowdhury, S., and Lelieveld, J. (2020). COVID-19 lockdowns cause global air pollution declines. Proc. Nat. Acad. Sci. 117, 18984–18990. doi: 10.1073/pnas.2006853117
Verma, A., Harsha, V., and Subramanian, G. H. (2021). Evolution of urban transportation policies in India: a review and analysis. Transp. Dev. Econ. 7, 1–15. doi: 10.1007/s40890-021-00136-1
WHO (2016). Ambient Air Pollution: A Global Assessment of Exposure and Burden of Disease . Available online at: https://www.who.int/phe/publications/air-pollution-global-assessment/en/ (accessed January 21, 2021).
WHO (2021). WHO Global Air Quality Guidelines: Particulate Matter (PM2. 5 and PM10), Ozone, Nitrogen Dioxide, Sulfur Dioxide and Carbon Monoxide: Executive Summary .
Wiebe, K., Robinson, S., and Cattaneo, A. (2019). Climate change, agriculture and food security: impacts and the potential for adaptation and mitigation. Sustain. Food Agricult. 2, 55–74. doi: 10.1016/B978-0-12-812134-4.00004-2
Wilk, J., Jonsson, A. C., Rydhagen, B., Rani, A., and Kumar, A. (2018). The perspectives of the urban poor in climate vulnerability assessments-The case of Kota, India. Urban Clim. 24, 633–642. doi: 10.1016/j.uclim.2017.08.004
Woodruff, J. D., Irish, J. L., and Camargo, S. J. (2013). Coastal flooding by tropical cyclones and sea-level rise. Nature 504, 44–52. doi: 10.1038/nature12855
Workie, T. G., and Debella, H. J. (2018). Climate change and its effects on vegetation phenology across ecoregions of Ethiopia. Global Ecol. Conserv. 13, e00366. doi: 10.1016/j.gecco.2017.e00366
World Bank (2014). The World Bank Annual Report 2014 . doi: 10.1596/978-1-4648-0245-4
World Bank (2021). World Bank Open Data . Available online at: worldbank.org (accessed September 10, 2023).
Xiang, X., Li, Q., Khan, S., and Khalaf, O. I. (2021). Urban water resource management for sustainable environment planning using artificial intelligence techniques. Environ. Impact Assess. Rev. 86, 106515. doi: 10.1016/j.eiar.2020.106515
Yan, Z. W., Wang, J., Xia, J. J., and Feng, J. M. (2016). Review of recent studies of the climatic effects of urbanization in China. Adv. Clim. Change Res. 7, 154–168. doi: 10.1016/j.accre.2016.09.003
Yang, A. S., Juan, Y. H., Wen, C. Y., and Chang, C. J. (2017). Numerical simulation of cooling effect of vegetation enhancement in a subtropical urban park. Appl. Energy 192, 178–200. doi: 10.1016/j.apenergy.2017.01.079
Yenneti, K., Tripathi, S., Wei, Y. D., Chen, W., and Joshi, G. (2016). The truly disadvantaged? Assessing social vulnerability to climate change in urban India. Habitat Int. 56, 124–135. doi: 10.1016/j.habitatint.2016.05.001
Zakaria, A., Azumah, S. B., Appiah-Twumasi, M., and Dagunga, G. (2020). Adoption of climate-smart agricultural practices among farm households in Ghana: the role of farmer participation in training programmes. Technol. Soc. 63, 101338. doi: 10.1016/j.techsoc.2020.101338
Zaveri, E., Russ, J., and Damania, R. (2020). Rainfall anomalies are a significant driver of cropland expansion. Proc. Nat. Acad. Sci. 117, 10225–10233. doi: 10.1073/pnas.1910719117
Zayan, S. A. (2019). “Impact of climate change on plant diseases and IPM strategies,” in Plant Diseases-Current Threats and Management Trends. London, UK: IntechOpen.
Keywords: urbanization and development, climate change, human health, sustainable development, India
Citation: Hussain S, Hussain E, Saxena P, Sharma A, Thathola P and Sonwani S (2024) Navigating the impact of climate change in India: a perspective on climate action (SDG13) and sustainable cities and communities (SDG11). Front. Sustain. Cities 5:1308684. doi: 10.3389/frsc.2023.1308684
Received: 06 October 2023; Accepted: 24 November 2023; Published: 11 January 2024.
Reviewed by:
Copyright © 2024 Hussain, Hussain, Saxena, Sharma, Thathola and Sonwani. This is an open-access article distributed under the terms of the Creative Commons Attribution License (CC BY) . The use, distribution or reproduction in other forums is permitted, provided the original author(s) and the copyright owner(s) are credited and that the original publication in this journal is cited, in accordance with accepted academic practice. No use, distribution or reproduction is permitted which does not comply with these terms.
*Correspondence: Saurabh Sonwani, sonwani.s19@gmail.com ; sonwani@zh.du.ac.in
This article is part of the Research Topic
Towards 2030: A Sustainable Cities Perspective on Achieving Sustainable Development Goal 13 – Climate Action
Thank you for visiting nature.com. You are using a browser version with limited support for CSS. To obtain the best experience, we recommend you use a more up to date browser (or turn off compatibility mode in Internet Explorer). In the meantime, to ensure continued support, we are displaying the site without styles and JavaScript.
- View all journals
- Explore content
- About the journal
- Publish with us
- Sign up for alerts
- 23 May 2022
Climate change made South Asian heatwave 30 times more likely
- Jude Coleman
You can also search for this author in PubMed Google Scholar
Human-induced climate change made the deadly heatwave that gripped India and Pakistan in March and April 30 times more likely, according to a rapid analysis of the event . Temperatures began rising earlier than usual in March, shattering records and claiming at least 90 lives. The prolonged heat has yet to subside.
Access options
Access Nature and 54 other Nature Portfolio journals
Get Nature+, our best-value online-access subscription
24,99 € / 30 days
cancel any time
Subscribe to this journal
Receive 51 print issues and online access
185,98 € per year
only 3,65 € per issue
Rent or buy this article
Prices vary by article type
Prices may be subject to local taxes which are calculated during checkout
doi: https://doi.org/10.1038/d41586-022-01444-1
Reprints and permissions
Related Articles
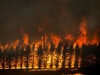
- Climate sciences
- Climate change
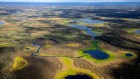
Epic blazes threaten Arctic permafrost. Can fire-fighters save it?
News 29 APR 24
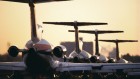
Air-travel climate-change emissions detailed for nearly 200 nations
Research Highlight 25 APR 24
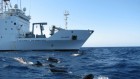
NATO is boosting AI and climate research as scientific diplomacy remains on ice
News Explainer 25 APR 24
W2 Professorship with tenure track to W3 in Animal Husbandry (f/m/d)
The Faculty of Agricultural Sciences at the University of Göttingen invites applications for a temporary professorship with civil servant status (g...
Göttingen (Stadt), Niedersachsen (DE)
Georg-August-Universität Göttingen
W1 professorship for „Tissue Aspects of Immunity and Inflammation“
Kiel University (CAU) and the University of Lübeck (UzL) are striving to increase the proportion of qualified female scientists in research and tea...
University of Luebeck
W1 professorship for "Bioinformatics and artificial intelligence that preserve privacy"
Kiel, Schleswig-Holstein (DE)
Universität Kiel - Medizinische Fakultät
W1 professorship for "Central Metabolic Inflammation“
W1 professorship for "Congenital and adaptive lymphocyte regulation"
Sign up for the Nature Briefing newsletter — what matters in science, free to your inbox daily.
Quick links
- Explore articles by subject
- Guide to authors
- Editorial policies
Advertisement
Climate Change: A Case Study Over India
- Published: November 1998
- Volume 61 , pages 9–18, ( 1998 )
Cite this article
- A. K. Sahai 1
481 Accesses
12 Citations
Explore all metrics
A brief account of various causes of climate change in recent decades and climate change trends in the Indian region is presented. It is of great importance to determine the influence of human activities on the likely climate change during recent decades. Local temperature is one of the major climatic elements to record the changes in the atmospheric environment caused by industrialization and urbanization. It is mentioned in the literature that there is either a cooling tendency or cessation of warming after the late 1950s at most of the Indian industrial cities. A case study of Nagpur, a centrally located city in India, is done to understand and the possible cause of cooling. Nagpur is the only city in India for which a long-term record of temperature, for urban (Mayo Hospital) and relatively suburban (Sonegaon Airport) area, is available. The study of the diurnal asymmetry in maximum and minimum temperatures indicates that the role of suspended particulate matter dominates over that of increasing greenhouse gases.
This is a preview of subscription content, log in via an institution to check access.
Access this article
Price includes VAT (Russian Federation)
Instant access to the full article PDF.
Rent this article via DeepDyve
Institutional subscriptions
Similar content being viewed by others
A review of recent advances in research on extreme heat events, the impact of urbanization and climate change on urban temperatures: a systematic review.
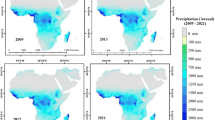
Global Warming Status in the African Continent: Sources, Challenges, Policies, and Future Direction
Author information, authors and affiliations.
Indian Institute of Tropical Meteorology, Pune, India, , , , , , IN
A. K. Sahai
You can also search for this author in PubMed Google Scholar
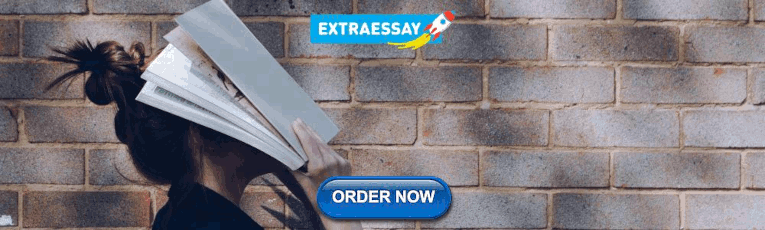
Additional information
Received April 15, 1998
Rights and permissions
Reprints and permissions
About this article
Sahai, A. Climate Change: A Case Study Over India. Theor Appl Climatol 61 , 9–18 (1998). https://doi.org/10.1007/s007040050047
Download citation
Issue Date : November 1998
DOI : https://doi.org/10.1007/s007040050047
Share this article
Anyone you share the following link with will be able to read this content:
Sorry, a shareable link is not currently available for this article.
Provided by the Springer Nature SharedIt content-sharing initiative
- Climate Change
- Human Activity
- Recent Decade
- Minimum Temperature
- Find a journal
- Publish with us
- Track your research
India holds the key to hitting global climate change targets. Here’s why
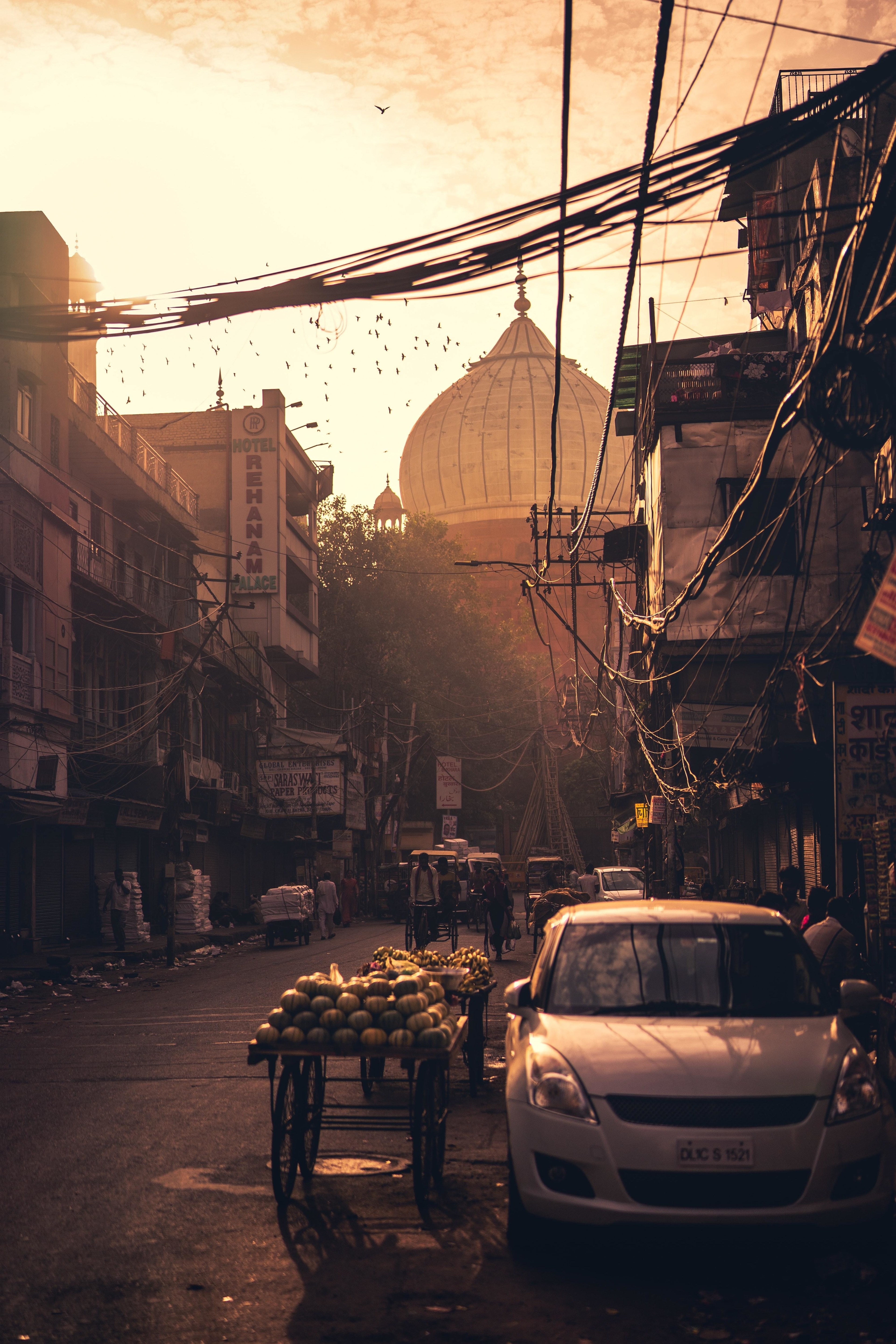
India has been at the forefront of driving global action on climate change. Image: Unsplash: Dewang Gupta
.chakra .wef-1c7l3mo{-webkit-transition:all 0.15s ease-out;transition:all 0.15s ease-out;cursor:pointer;-webkit-text-decoration:none;text-decoration:none;outline:none;color:inherit;}.chakra .wef-1c7l3mo:hover,.chakra .wef-1c7l3mo[data-hover]{-webkit-text-decoration:underline;text-decoration:underline;}.chakra .wef-1c7l3mo:focus,.chakra .wef-1c7l3mo[data-focus]{box-shadow:0 0 0 3px rgba(168,203,251,0.5);} Viraj Mehta
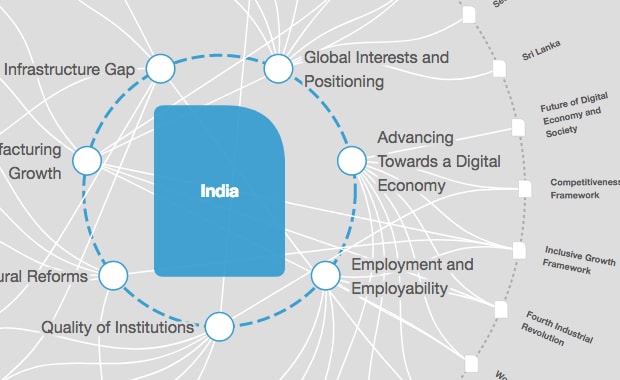
.chakra .wef-9dduvl{margin-top:16px;margin-bottom:16px;line-height:1.388;font-size:1.25rem;}@media screen and (min-width:56.5rem){.chakra .wef-9dduvl{font-size:1.125rem;}} Explore and monitor how .chakra .wef-15eoq1r{margin-top:16px;margin-bottom:16px;line-height:1.388;font-size:1.25rem;color:#F7DB5E;}@media screen and (min-width:56.5rem){.chakra .wef-15eoq1r{font-size:1.125rem;}} India is affecting economies, industries and global issues
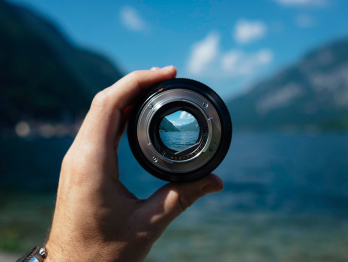
.chakra .wef-1nk5u5d{margin-top:16px;margin-bottom:16px;line-height:1.388;color:#2846F8;font-size:1.25rem;}@media screen and (min-width:56.5rem){.chakra .wef-1nk5u5d{font-size:1.125rem;}} Get involved with our crowdsourced digital platform to deliver impact at scale
Stay up to date:, davos agenda.
Listen to the article
- India has sizeable and growing energy needs, and with this, comes the prospect of spiralling greenhouse gas emissions (GHGs).
- The country has made huge strides in improving energy access, but needs to continue to develop the energy network and diversify its fuel mix.
- The country’s path to decarbonization could be a net-positive one if it turns to low- and no-emissions technologies.
- Any successful models it develops can be replicated in other economies, producing a beneficial effect for billions of people.
Some time in 2023, likely mid-April, India will surpass China as being the country with the largest population. It is a timely reminder of the growing influence that India and its activities exert on the rest of the world, and not just because of its size.
With such a large – still industrializing – country come sizeable energy needs, and with this, the prospect of spiralling greenhouse gas emissions (GHGs). India is grappling with the issue of trying to quickly develop equitable economic growth to meet the changing needs and lifestyle aspirations of its 1.4 billion people, while also hitting national and global climate change targets.
It should come as little surprise, therefore, that India has been at the forefront of driving global action on climate change. It has used indigenous technology to optimize its resources and promoted green energy to reduce carbon emissions; Prime Minister Narendra Modi’s announcement to establish a National Hydrogen Mission is noteworthy. Alongside this, India has co-founded the International Solar Alliance (ISA) with France, and in doing so, is leading the global movement towards solar power, with a focus on promoting energy access and transition. Already, the ISA has 110 member countries and is pursuing nine programmes promoting 10GW of off-grid and grid-connected solar projects in developing countries.
India’s pledge to reach net-zero emissions by 2070 was one of the most important announcements at COP26. In line with the prime minister’s statement, the federal government recently approved India’s Updated Nationally Determined Contribution (NDC), which translates the COP26 announcements into enhanced climate targets. It marks a major step in achieving India’s long-term goal of reaching net zero by 2070.
Have you read?
Indigenous leadership is the key to unlocking value in nature-based solutions, why india's new climate mission is focused on sustainable lifestyles, decarbonization and carbon capture could solve india’s energy shortage, without fossil fuels, here’s how india became a global clean energy powerhouse.
Towards the end of 2022 and following the 75th year of its independence, India took on the mantle of G20 leadership. Its leadership slogan ‘one earth, one family, one future’, is particularly apt, underlining how inextricably linked humanity is, not least in suffering and addressing the effects of the changing climate. During 2022, extreme weather events were recorded in the country during 80% of the year , underlining how much India is already suffering the effects of climate change.
Reflecting this, energy and climate change mitigation are among India’s priorities during its G20 presidency. Specifically, India is expected to focus on climate finance, energy security and green hydrogen, pushing for the provision of finance and technology as critical enablers for achieving the Paris Agreement climate goals.
The G20 presidency places India on the global stage and allows it to establish its priorities and narratives within the international agenda. This makes 2023 a year to watch: How will India continue to fuel its growing energy needs and what decisions will it take about its energy transition?
The Fostering Effective Energy Transition 2023 report showed that after a decade of progress, the global energy transition has plateaued amid the global energy crisis and geopolitical volatilities.
The World Economic Forum’s Centre for Energy and Materials is driving the transition to a “fit for 2050” energy system. It is a cross-industry platform building new coalitions and delivering insights required for a sustainable, secure and just energy future.
Learn more about our impact:
- Clean energy in emerging economies: We are advancing country-specific renewable energy finance solutions for four of the biggest emerging and developing economies : India, Brazil, Nigeria and Indonesia. In the latter, a new solar and battery initiative is bringing 15MW of clean energy to the East Sumba region – enough to power 4,000 homes and avoid 5.5KtCO₂ yearly emissions.
- Energy Transition Index: We have measured the progress of 120 countries on the performance of their energy systems, enabling policymakers and businesses to identify the necessary actions for the energy transition.
- Mining and metals blockchain : We released a proof of concept to trace emissions across the value chain using blockchain technology, helping accelerate global action for country-specific financing solutions.
- Clean power and electrification: We are accelerating the adoption of clean power and electric solutions in the next decade to help increase clean energy consumption threefold by 2030.
Want to know more about our centre’s impact or get involved? Contact us .
The country has made huge strides in improving energy access during the past two decades, with near-universal household access achieved in 2019 . Its challenge now is to continue to develop the energy network and diversify its fuel mix to meet growing demand. The situation presents India with the opportunity to take bold action, putting it on a path to realizing strong, equitable, shared growth, while also averting the worst effects of the changing climate.
As the situation stands, however, India’s energy mix is carbon intensive. Coal is its primary source of fuel, accounting for approximately 70% of electricity generation , and the country also powers much of its transport through oil. As a result, India is the world’s third-largest emitter of CO2, despite low per capita CO2 emissions. Unlike developed nations with mature GHG infrastructure, India has yet to build a lot of its GHG inventory .
This suggests a need for the country to significantly reduce its reliance on fossil fuels. In the interim, it must make heavy investment into methods to combat the emissions problem, for example with implementing cleaner operations, stripping emissions of the worst of the GHGs, and looking at carbon capture.
The good news is that the country’s path to decarbonization could, however, be a net-positive one. India has a unique opportunity to skip this journey by turning to low- and no-emissions technologies. Already India is the world’s third-largest producer of solar energy and enjoys the lowest renewables costs. Plans to increase renewable energy as part of the mix remain in place, and India is pioneering green hydrogen (hydrogen made using renewable energy).
Mission 2070: A Green New Deal for a Net Zero India
Given this fertile situation, research has pointed to the benefits India would reap from pursuing a Green New Deal. It is estimated that by greening its economy, India could leverage green growth to add $1 trillion to its GDP by 2030 and a staggering $15 trillion by 2070. Additionally, such a transformation could create 50 million jobs .
The World Economic Forum remains committed to support India in its vision and efforts to drive climate action and environment sustainability, during and beyond its G20 presidency. During the Forum’s Annual Meeting 2022, the Forum launched the Alliance of CEO Climate Leaders India , a high-level platform aimed at supporting and accelerating the country’s climate action and green transition efforts. The alliance’s members – India’s leading business voices – will, at the Annual Meeting 2023, agree priorities, set targets and announce workstreams and timelines. The Forum is also contributing to the country’s climate and land restoration goals through its Trillion Tree platform, 1t.org. The platform is launching a coalition in India, which aims to bring together the public and private sectors, and civil society to support forest conservation and restoration efforts.
The G20 presidency offers India the opportunity to shape the climate and energy transition agenda at a global level. Reflecting its sheer size and diversity, any successful models it develops can be replicated in other economies, producing a beneficial effect for billions of people. India can establish itself as a role model through its response to these opportunities, potentially having a resounding impact on our collective future.
This article originally appeared in the Hindu Business Line .
Don't miss any update on this topic
Create a free account and access your personalized content collection with our latest publications and analyses.
License and Republishing
World Economic Forum articles may be republished in accordance with the Creative Commons Attribution-NonCommercial-NoDerivatives 4.0 International Public License, and in accordance with our Terms of Use.
The views expressed in this article are those of the author alone and not the World Economic Forum.
Related topics:
The agenda .chakra .wef-n7bacu{margin-top:16px;margin-bottom:16px;line-height:1.388;font-weight:400;} weekly.
A weekly update of the most important issues driving the global agenda
.chakra .wef-1dtnjt5{display:-webkit-box;display:-webkit-flex;display:-ms-flexbox;display:flex;-webkit-align-items:center;-webkit-box-align:center;-ms-flex-align:center;align-items:center;-webkit-flex-wrap:wrap;-ms-flex-wrap:wrap;flex-wrap:wrap;} More on Forum Institutional .chakra .wef-nr1rr4{display:-webkit-inline-box;display:-webkit-inline-flex;display:-ms-inline-flexbox;display:inline-flex;white-space:normal;vertical-align:middle;text-transform:uppercase;font-size:0.75rem;border-radius:0.25rem;font-weight:700;-webkit-align-items:center;-webkit-box-align:center;-ms-flex-align:center;align-items:center;line-height:1.2;-webkit-letter-spacing:1.25px;-moz-letter-spacing:1.25px;-ms-letter-spacing:1.25px;letter-spacing:1.25px;background:none;padding:0px;color:#B3B3B3;-webkit-box-decoration-break:clone;box-decoration-break:clone;-webkit-box-decoration-break:clone;}@media screen and (min-width:37.5rem){.chakra .wef-nr1rr4{font-size:0.875rem;}}@media screen and (min-width:56.5rem){.chakra .wef-nr1rr4{font-size:1rem;}} See all
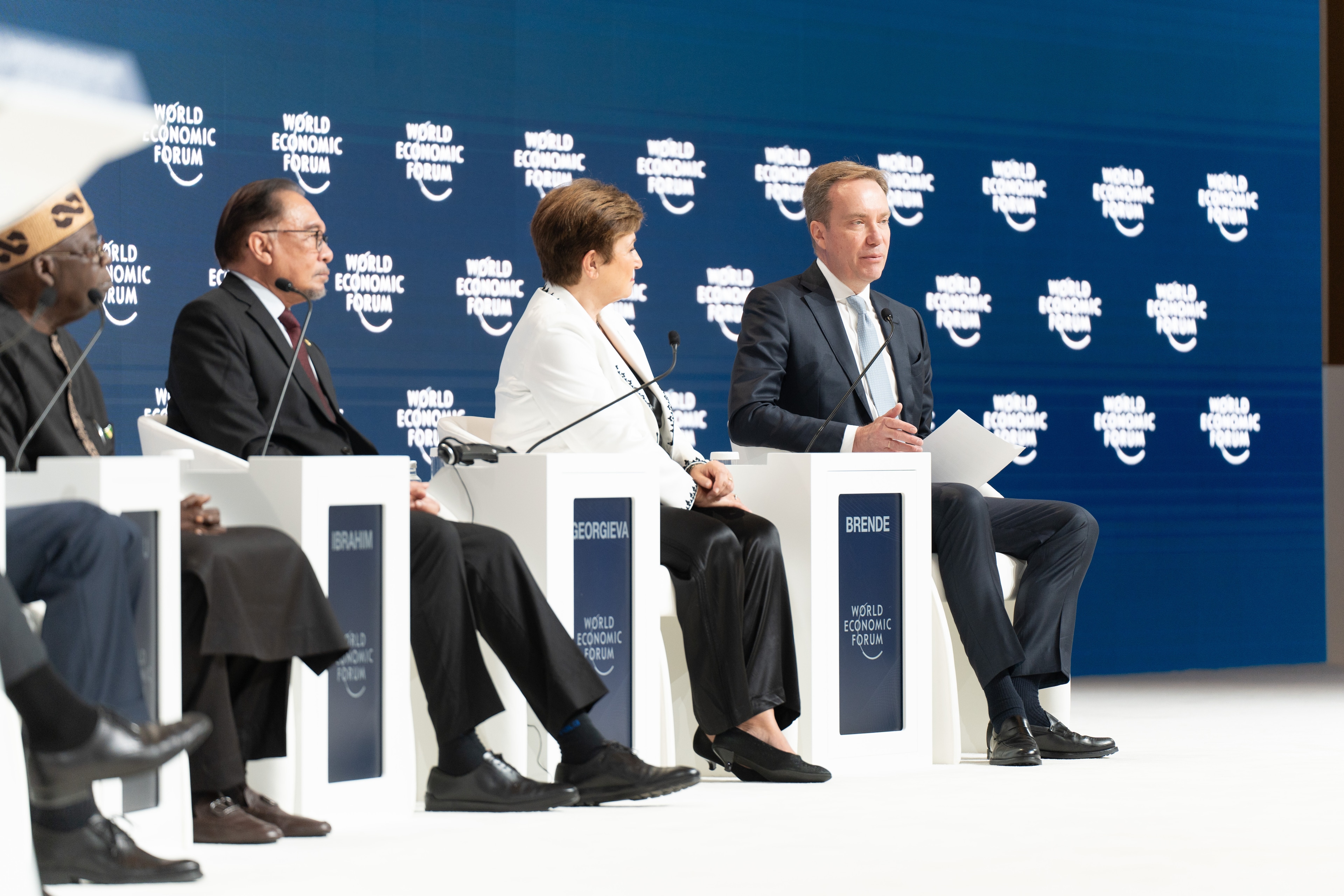
Day 2 #SpecialMeeting24: Key insights and what to know
Gayle Markovitz
April 28, 2024
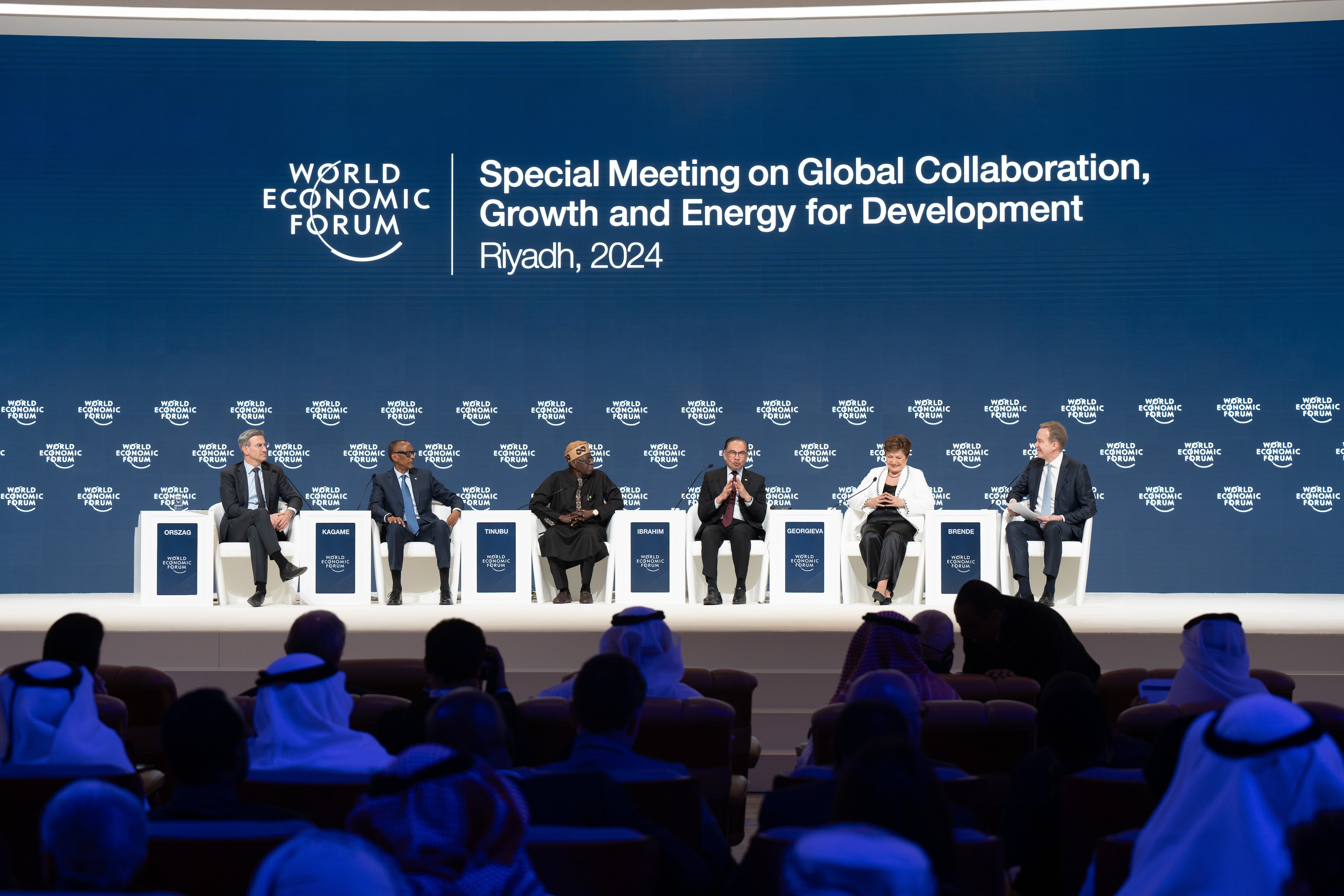
Day 1 #SpecialMeeting24: Key insights and what just happened
April 27, 2024
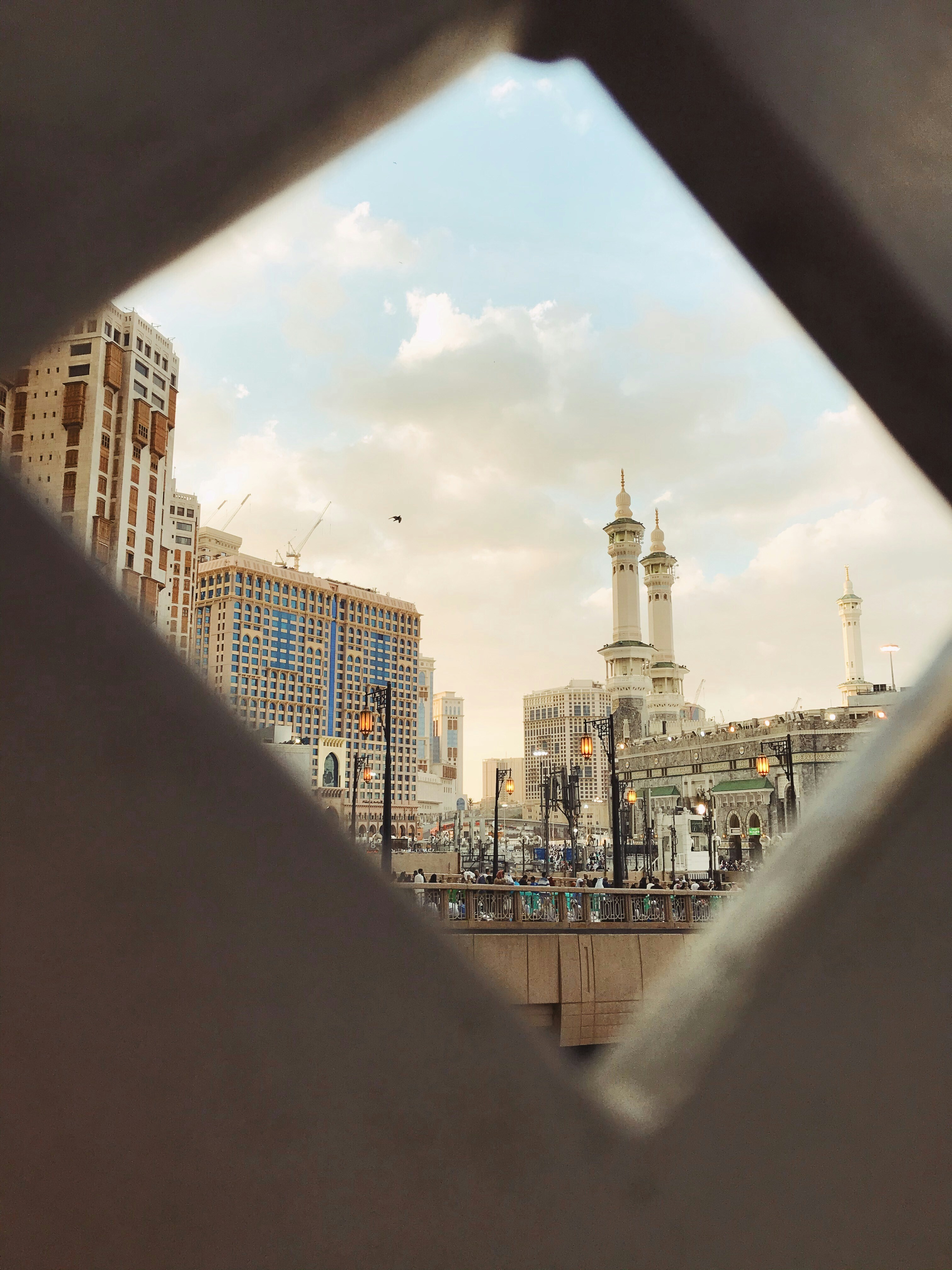
#SpecialMeeting24: What to know about the programme and who's coming
Mirek Dušek and Maroun Kairouz
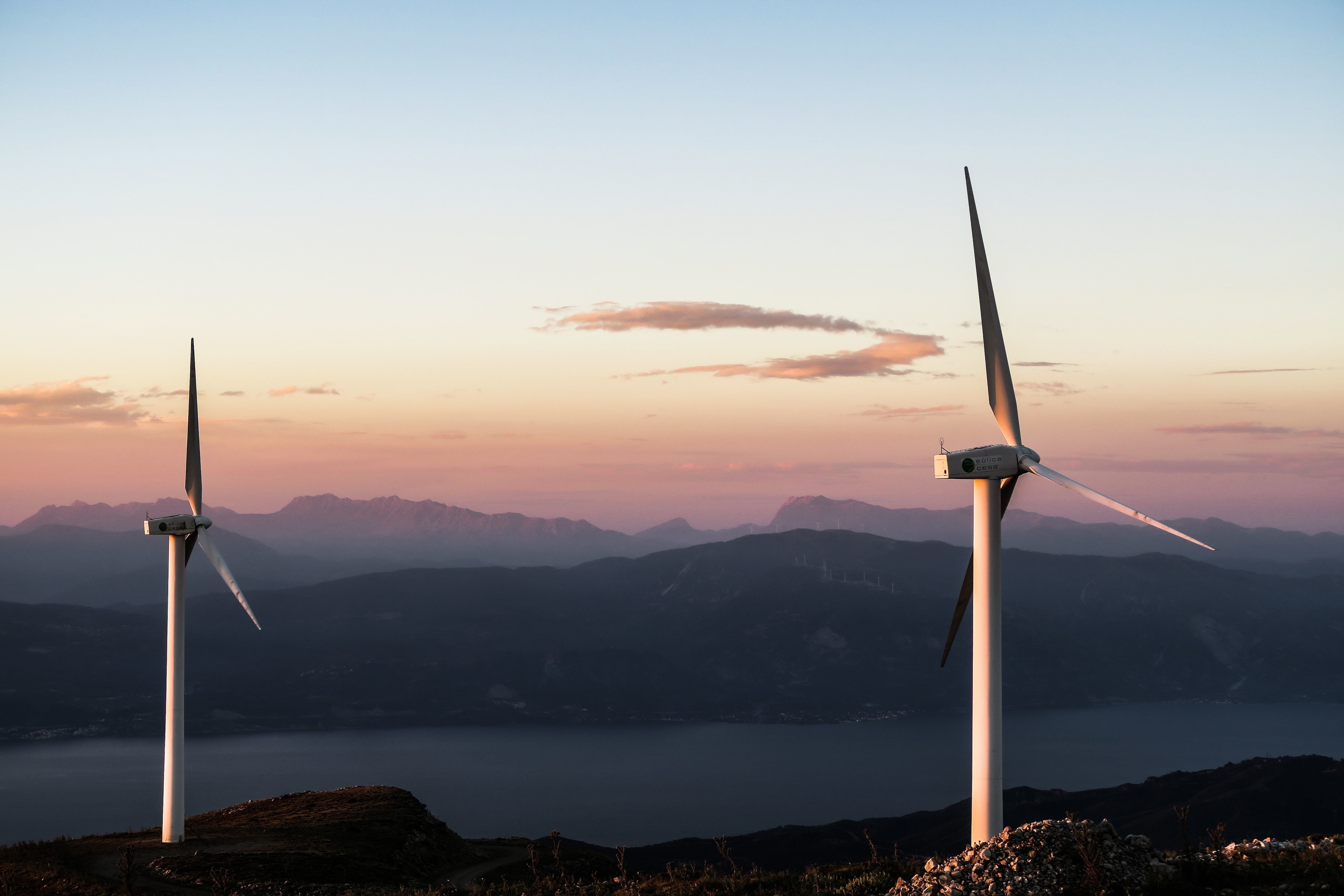
Climate finance: What are debt-for-nature swaps and how can they help countries?
Kate Whiting
April 26, 2024
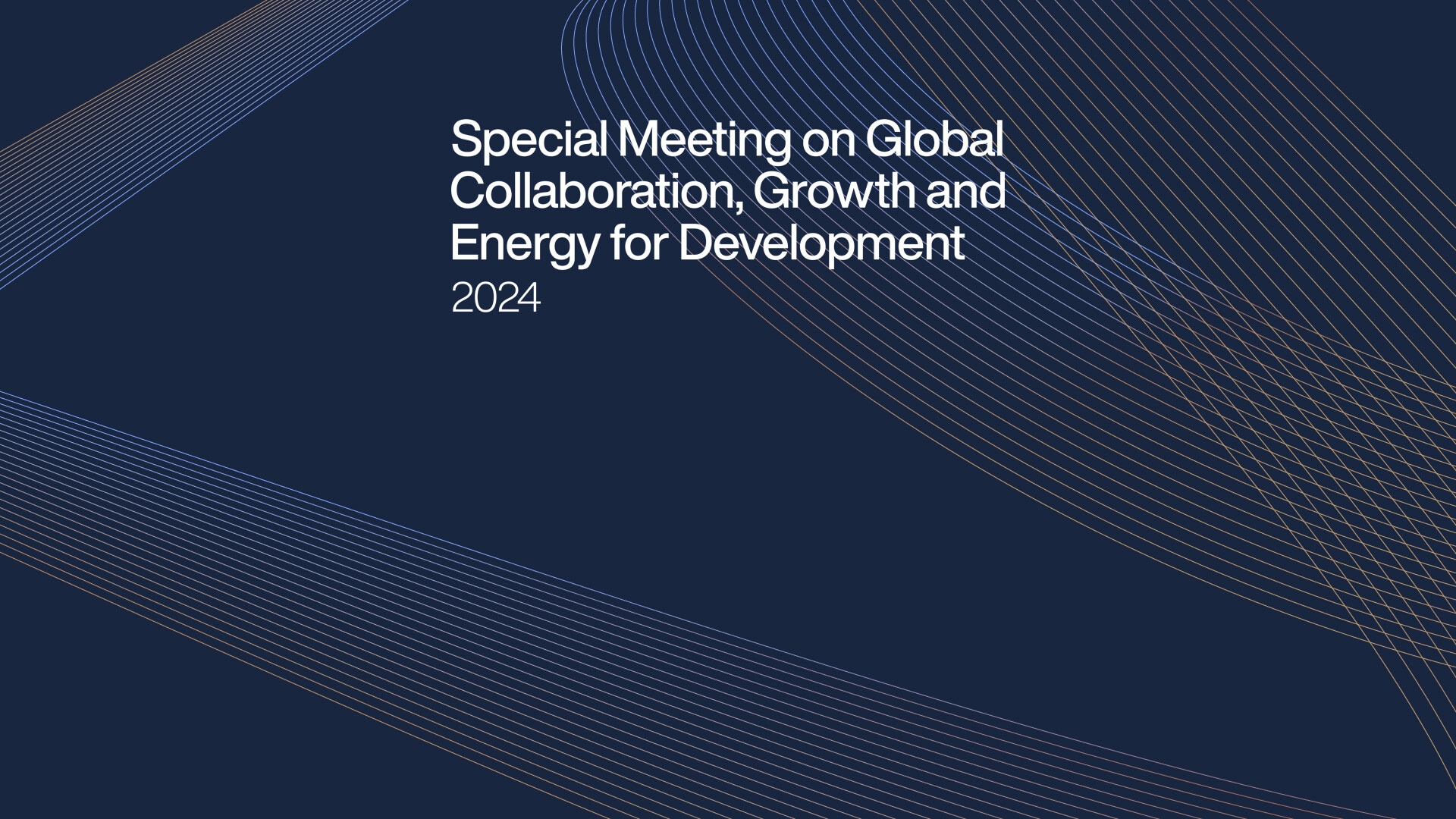
What to expect at the Special Meeting on Global Collaboration, Growth and Energy for Development
Spencer Feingold and Gayle Markovitz
April 19, 2024
From 'Quit-Tok' to proximity bias, here are 11 buzzwords from the world of hybrid work
April 17, 2024

An official website of the United States government
The .gov means it’s official. Federal government websites often end in .gov or .mil. Before sharing sensitive information, make sure you’re on a federal government site.
The site is secure. The https:// ensures that you are connecting to the official website and that any information you provide is encrypted and transmitted securely.
- Publications
- Account settings
Preview improvements coming to the PMC website in October 2024. Learn More or Try it out now .
- Advanced Search
- Journal List
- Environ Health Perspect
- v.119(6); 2011 Jun

Impacts of Climate Change on Public Health in India: Future Research Directions
Kathleen f. bush.
1 Department of Environmental Health Sciences, School of Public Health, University of Michigan, Ann Arbor, Michigan, USA
George Luber
2 National Center for Environmental Health, Centers for Disease Control and Prevention, Atlanta, Georgia, USA
S. Rani Kotha
3 University of Michigan Center for Global Health, Ann Arbor, Michigan, USA
R.S. Dhaliwal
4 Indian Council of Medical Research, New Delhi, India
Vikas Kapil
Mercedes pascual.
5 Department of Evolutionary and Ecological Biology, University of Michigan, Ann Arbor, Michigan, USA
6 Howard Hughes Medical Institute, Chevy Chase, Maryland, USA
Daniel G. Brown
7 School of Natural Resources and Environment, University of Michigan, Ann Arbor, Michigan, USA
Howard Frumkin
8 University of Washington School of Public Health, Seattle, Washington, USA
R.C. Dhiman
9 National Institute of Malaria Research, New Delhi, India
Jeremy Hess
Mark l. wilson.
10 Department of Epidemiology, School of Public Health, University of Michigan, Ann Arbor, Michigan, USA
Kalpana Balakrishnan
11 Department of Environmental Health and Engineering, Sri Ramachandra University, Chennai, India
Joseph Eisenberg
Tanvir kaur, richard rood.
12 Department of Atmospheric, Oceanic and Space Sciences, University of Michigan, Ann Arbor, Michigan, USA
Stuart Batterman
Aley joseph, carina j. gronlund, arun agrawal.
Climate change and associated increases in climate variability will likely further exacerbate global health disparities. More research is needed, particularly in developing countries, to accurately predict the anticipated impacts and inform effective interventions.
Building on the information presented at the 2009 Joint Indo–U.S. Workshop on Climate Change and Health in Goa, India, we reviewed relevant literature and data, addressed gaps in knowledge, and identified priorities and strategies for future research in India.
The scope of the problem in India is enormous, based on the potential for climate change and variability to exacerbate endemic malaria, dengue, yellow fever, cholera, and chikungunya, as well as chronic diseases, particularly among the millions of people who already experience poor sanitation, pollution, malnutrition, and a shortage of drinking water. Ongoing efforts to study these risks were discussed but remain scant. A universal theme of the recommendations developed was the importance of improving the surveillance, monitoring, and integration of meteorological, environmental, geospatial, and health data while working in parallel to implement adaptation strategies.
Conclusions
It will be critical for India to invest in improvements in information infrastructure that are innovative and that promote interdisciplinary collaborations while embarking on adaptation strategies. This will require unprecedented levels of collaboration across diverse institutions in India and abroad. The data can be used in research on the likely impacts of climate change on health that reflect India’s diverse climates and populations. Local human and technical capacities for risk communication and promoting adaptive behavior must also be enhanced.
Climate Change and Human Health
Although low- and middle-income countries are responsible for only a small percentage of global greenhouse gas emissions, the adverse health effects associated with climate change will likely fall disproportionately on their populations. This inequity will further exacerbate global health disparities ( McMichael et al. 2003 ; Patz and Olson 2006 ; Patz et al. 2007 ; Wiley and Gostin 2009 ). High-risk areas include those already experiencing a scarcity of resources, environmental degradation, high rates of infectious disease, weak infrastructure, and overpopulation ( Patz et al. 2005 ; Wiley and Gostin 2009 ). In particular, tropical regions will experience significant changes in human–pathogen relationships because of climate change ( Sattenspiel 2000 ). Changing temperatures and precipitation patterns linked to climate change will further affect health by changing the ecology of various vector-borne diseases, such as malaria, dengue, chikungunya, Japanese encephalitis, kala-azar, and filariasis ( Bhattacharya et al. 2006 ; Dhiman et al. 2008 ). Vulnerable populations include the elderly, children, urban populations, and the poor ( Ebi and Paulson 2010 ; O’Neill and Ebi 2009 ).
The goals of this report are to briefly summarize relevant literature and highlight the enormous challenges and opportunities for innovative research, with a particular focus on India. Such research is needed to pave the way for unique and pioneering solutions that can improve public health in the face of increasing climate variability. Therefore, we review the current state of the science relevant to the 2009 Joint Indo–U.S. Workshop on Climate Change and Health that was held in Goa, India, and then discuss the observed relationships between climate variability and human health, specifically in relation to the Indian subcontinent, highlighting future research directions.
Potential health impacts discussed at the Goa workshop fell into three categories: heat stress and air pollution, waterborne disease, and vector-borne disease focusing on malaria. Additional crosscutting sessions covered climate modeling and predictions for India, adaptation and vulnerability, surveillance and early warning systems, integration of spatial analysis, and bridging policy and science. We acknowledge that the potential physical and social impacts of climate change in India will likely be diverse, and that many additional important factors were not covered in our workshop, such as food yields, malnutrition, child growth, river flow, monsoon rain patterns, and freshwater availability. Nevertheless, we believe the Goa workshop served to target many of the major public health concerns associated with climate change and began the process of conceptualizing research needs and approaches that are integrative and achievable in low- and middle-income countries.
Impacts in India
The 2009 joint indo-u.s. workshop on climate change and health.
The workshop was held in Goa, India, on 30 August through 2 September 2009; it was cosponsored by the University of Michigan’s Center for Global Health, the U.S. Centers for Disease Control and Prevention’s National Center for Environmental Health, and the Indian Council of Medical Research. Scientists from the cosponsoring institutions, along with other partners from academia, government, and nongovernmental organizations, met under the auspices of the existing Indo-U.S. Collaboration in Environmental and Occupational Health to discuss the current state of the science, identify gaps in understanding, and outline future research directions related to the human health effects of climate change in India. The focus was prediction and prevention in India, and discussions touched on the tremendous opportunities and significant challenges associated with designing, initiating, and conducting research, as well as pursuing related public health programming to improve public health infrastructure in the face of climate change.
The scope of the problem and current research
Poverty and baseline vulnerability.
Many of the predicted effects of climate change are likely to become a reality in India. India is very diverse, geographically, climatically, and culturally ( Figure 1A ). It represents one-sixth of the world’s population, supported on 1/50 of the world’s land and 1/25 of the world’s water ( Singh et al. 2010 ). With its huge and increasing population (~ 1.2 billion) and rate of urbanization, India is undergoing enormous change; climate change poses an overwhelming stressor that will magnify existing health threats. A greater understanding of the relationship between climate variability and human health in a country such as India could aid in the development of new prevention strategies and early warning systems, with implications throughout the developing world. Future studies must work to more explicitly define the relationship between climate variability and emerging and reemerging infectious diseases such as dengue, yellow fever, cholera, and the chikungunya virus ( Shope 1991 ), as well as chronic diseases related to cardiovascular and respiratory illness, asthma, and diabetes. Millions of people below the poverty line and those in rural areas represent high-risk populations who are exposed to myriad health risks, including poor sanitation, pollution, malnutrition, and a constant shortage of clean drinking water. However, as awareness and public health infrastructure increase, the burden of climate-related disease may be negated ( Dhiman et al. 2010 ).

Interactions between climate and health in India. ( A ) Climate zones in India based on the Köppen classification that demonstrates the diversity of climates that exist in India [adapted from WikiProject India Maps and licensed under Creative Commons Attribution-Share Alike 3.0 ( http://creativecommons.org/licenses/by-sa/3.0/ )]. ( B ) State-specific estimates of cases of diarrheal disease across India in 2006 (adapted from Mandal 2008 ). ( C and D ) Regional estimates of malaria prevalence across India in 2002 ( C ) and regions in India where the prevalence of malaria is predicted to increase because of changes in climate ( D ) (adapted from Bhattacharya et al. 2006 ).
Waterborne infectious disease
The burden of waterborne disease in India is enormous ( Figure 1B ). However, estimates vary widely because of a lack of reporting, poor surveillance, and minimal data infrastructure. A report from the Ministry of Health and Family Welfare estimates that nearly 40 million people are affected by waterborne disease every year that places a large burden on both the health sector and the economic sector. As a consequence, approximately 73 million workdays or US$600 million are lost each year ( Mandal 2008 ). Although the World Health Organization (WHO) estimates that 900,000 Indians die each year from drinking contaminated water and breathing polluted air ( WHO and UNICEF 2000 ), the Indian Ministry of Health estimates 1.5 million deaths annually among 0- to 5-year-old children. Cholera provides another example, with approximately 5 million cases reported by WHO each year; however, this estimate is thought to be a gross underestimation of the true burden of cholera because of a lack of surveillance and underreporting on the Indian subcontinent ( Zuckerman et al. 2007 ). Approximately 73% of the rural population in India does not have proper water disinfection, and 74% do not have sanitary toilets ( International Institute for Population Sciences and Macro International 2007 ). Freshwater availability in India is also a concern; available water is expected to decrease from 1,820 m 3 per capita to < 1,000 m 3 by 2025 in response to the combined effects of population growth and climate change ( Intergovernmental Panel on Climate Change 2007 ).
Research in this area must be both temporally and spatially specific. Furthermore, it requires local monitoring of the appropriate climate and disease variables ( Patz et al. 2002 ) because underreporting impedes the development of effective prevention strategies. It is critical to build a data infrastructure and conduct such research in India so that region-specific models based on climate and health can be developed. A systems approach focusing on health outcomes is critical to the success of future research in this area ( Batterman et al. 2009 ). As prediction models evolve, region-specific action plans and adaptation strategies can be developed.
Heat stress and air pollution
The summer of 2010 was the hottest summer on record in India, with temperatures approaching 50°C (122°F); the effects were far-reaching, including hospitalization because of heatstroke, suffering of livestock, and severe drought in some regions that affected health as well as agriculture ( Burke 2010 ). Research linking temperature and health effects in India is sparse. However, in a study of 12 international urban areas that included Delhi, McMichael et al. (2008) found a 3.94% [95% confidence interval (CI), 2.80–5.08%] increase in mortality for each 1°C increase above 29°C. Hajat et al. (2005) reported that individuals in the 0- to 14-year-old age group had greater vulnerability to temperature increases in Delhi than did those in the 15- to 64-year-old age group or in the ≥ 65-year-old age group. These findings are in direct contrast with results from cities in Europe and the United States that consistently identify the elderly as the more vulnerable age group. Hajat et al. (2005) also found that harvesting (whereby increases in mortality on one day are followed by substantial decreases in mortality in subsequent days) accounted for almost all temperature-related mortality in London, whereas in Delhi, the increase in mortality due to high temperatures was not followed by an immediate drop in mortality. This suggests that in Delhi, individuals who died on days with higher temperatures were not already near death.
Limited work has been conducted on the combined effects of weather, climate variability, and increased air pollution in India ( Agarwal et al. 2006 ; Karar et al. 2006 ). One study that investigated the effects of air pollution on respiratory disease found that emergency department visits increased by approximately 20% because of high levels of pollutants in Delhi ( Pande et al. 2002 ). In a second study based in Chennai, India, Ghosh et al. (2010) concluded that short-term exposure to particulate matter ≤ 10 μm in aerodynamic diameter (PM 10 ) resulted in an estimated risk ratio of 1.0044 (95% CI, 1.002–1.007) per a 10 μg/m 3 increase in daily average concentrations; this risk estimate is comparable to similar estimates from other countries. An important contribution of this study, relevant to other low- and middle-income countries, was the development of new methods to address specific limitations of routinely collected data such as missing measurements and small footprints of air pollution monitors, but the link to temperature remains to be explored. Some work has been done on seasonal air quality monitoring ( Pulikesi et al. 2006 ); however, the relationship of temperature, ozone, and health requires further investigation ( Doherty et al. 2009 ). Indoor air pollution presents yet another major health threat, with 32% of deaths in South Asia attributable to the burning of solid fuels in poor, small, unventilated houses ( Smith 2000 ; WHO 2004 ). Whether these health risks will be exacerbated as a result of climate change is yet to be determined, but cobenefit interventions aimed at reducing the health impacts associated with indoor air pollution, decreasing the release of green house gases from the burning of solid fuel, and preventing deforestation by introducing alternative, more efficient stoves and fuels will have serious implications for health and society.
Vector-borne disease
India has approximately 2 million confirmed cases of malaria per year ( Kumar et al. 2007 ). Like most infectious diseases, prevalence varies by region ( Figure 1C,D ). Although WHO concludes that approximately 15,000 individuals die from malaria each year in India ( WHO 2008 ), a recent study by Dhingra et al. (2010) estimates approximately 200,000 malaria deaths per year in India before 70 years of age and 55,000 in early childhood. As Dhingra et al. (2010) suggest, accurate estimation of malaria mortality in India is difficult because correctly diagnosed episodes are successfully treated and do not result in death; in fatal cases without medical intervention, malaria is easily mistaken for some other life-threatening fever; and in most rural areas where death from malaria is common, proper medical attention at the time of death is uncommon. These challenges, which hold true in many developing countries, make it difficult to use hospital-based data to assess the association between climate variability and malaria, because disease burden may be vastly underestimated.
In India, 65% of malaria cases are reported from six regions (Orissa, Jharkhand, Madya Pradesh, Chattsgarh, West Bengal, and the North East). In Orissa, the disease has much more serious proportions than even in sub-Saharan Africa ( Narain 2008 ). A 2001 WHO report estimated the disability-adjusted life years lost because of all vector-borne diseases in the country to be 4.2 million, and malaria is believed to account for nearly half of this ( Dash et al. 2008 ). The emergence and rapid spread of drug-resistant strains of malaria further compound the problem. Chloroquine used to be the drug of choice for all kinds of malaria and was highly prescribed in India until 1973, when resistance was detected in Plasmodium falciparum . Chloroquine is no longer as effective, with increasing reports of Plasmodium vivax developing resistance ( Dash et al. 2008 ). In addition, the use of chloroquine, which selects against P. vivax , has allowed P. falciparum to become the dominant parasite ( Singh et al. 2004 ), a pattern with important epidemiological consequences, because it is the most virulent form of malaria in the region.
In arid and semiarid regions of India, where malaria is epidemic, rainfall variability has been shown to drive the interannual variability of the disease ( Akhtar and McMichael 1996 ; Bouma and van der Kaay 1994 ; Laneri et al. 2010 ) and was the basis of one of the first early-warning systems for the disease in this region. Evidence suggests that rainfall variability plays an important role and that a long-term trend in increasing temperature during the 20th century is sufficient to significantly increase the abundance of vectors ( Pascual et al. 2009 ). Monthly parasite incidence was positively correlated with temperature, precipitation, and humidity ( Devi and Jauhari 2006 ). The implications of this association as it relates to long-term climate change remain an important open question. For other regions of India, monsoonal rains have shown an increase in the frequency and magnitude of extreme rain events, whereas the frequency of moderate events has been decreasing, with no significant change in the mean in the last 50 years ( Goswami et al. 2006 ). Temperature plays a major role, especially at high altitudes, preventing epidemic malaria from spreading into the highest altitude regions. The consequences of climate change in highland regions is an important open question based on future temperature predictions in these regions (Beig G, unpublished data). Little is known about the influence of climate variability or climate change on the prevalence of malaria in Indian urban areas ( Kumar et al. 2007 ). The issue of urban malaria becomes even more important when considering the rapid expansion of urban and periurban environments, water storage techniques, and rising poverty levels.
The need for adaptation
Although adaptation to climate impacts has attracted substantial attention recently, the effectiveness of specific strategies in relation to greater resilience of public health systems remains underinvestigated. Adapting to climate change will be necessary and will occur at physiological, behavioral, social, institutional, and organizational scales. To take advantage of already ongoing adaptations for creating more effective public health responses to climate change impacts—especially for poor rural communities whose access to health care is extremely limited even in the current policy environment—developing a baseline understanding of the region-specific demographic, social, and ecological determinants of health will be necessary. In designing public health responses, factors that must be considered include the population’s age structure, socioeconomic profile, baseline prevalence of climate-sensitive diseases, public awareness of risk, the built environment, existing infrastructure, available public health services, and autonomous responses to climate impacts on health that households and communities might undertake by themselves ( McMichael 2004 ). Furthermore, adaptation strategies in response to climate variability and change must be designed on specific temporal and spatial scales relevant to India. Taking steps now to adjust to current climate variability and modifying existing programs to address the anticipated impacts of climate change will make future adaptation strategies more effective ( Ebi et al. 2006 ). The same changes may also aid in reaching additional environmental and social objectives, such as more equitable education, empowerment of women, and improved sanitation. These community-based initiatives should be complemented by government interventions. A variety of stakeholders, including those who will be affected most by climate change impacts, must be involved in the problem-solving process to enhance human and technical capacity across sectors at both local and national levels ( Agrawal 2009 ; Ebi and Semenza 2008 ). Failure to invest now will likely increase the severity of consequences in the future ( Haines et al. 2006 ).
Potential adaptation strategies in India could focus on controlling infectious diseases by removing vector breeding sites, reducing vector–human contact via improved housing, and coordinating monitoring of mosquitoes, pathogens, and disease burden. Another potential focus area for adaptation could be improving sanitation and drinking water by supporting inexpensive and effective water treatment and increasing rainwater harvesting, safe storage, and gray-water reuse. In some areas, the focus may shift to flood, heat wave, and emergency preparedness, including strategies to address the additional risks placed on displaced populations from these and other climate-sensitive hazards. One possible outcome could be the development of an integrated early warning system, emergency response plans, and refugee management plans, along with increased capacity to provide shelter, drinking water, sanitation, and sustainable agricultural products to the most vulnerable populations.
Current surveillance and data sources
Successful work in this area will require the health community to partner closely with climate scientists and development professionals to move beyond the assessment of climate variability and disease outcomes to predictive models accounting for climate change to facilitate targeted adaptation. Partnerships with both the government and nongovernment sector will also be necessary. An integrated disease surveillance system already exists under the director general of health services; any new work on climate change and health should be linked to the already existing system. The Energy and Resources Institute (TERI) in Delhi, India, is one example of such a group linking research and action by increasing awareness within India and sharing the “developing country” perspective on climate change with the rest of the world. Activities at TERI range from operating as a think tank at the local level to forging global alliances for collaborative research. Collaborative work is also being conducted at the National Institute of Malaria Research in partnership with Mercedes Pascual at the University of Michigan to assess the impacts of climate change on malaria and dengue at a national scale and to develop adaptation strategies. In addition, this same partnership is developing an evidence-based assessment of biophysical determinants affecting malaria in the northeastern states of India and a framework for malaria control under changing climate scenarios. Several other nongovernmental organizations are working on climate change in India, such as the Local Governments for Sustainability with a regional office in New Delhi; Resources for the Future, which is partnering closely with the Public Health Foundation of India; and Toxics Link, which is working on traditional environmental health with a new focus on climate change.
Retrospective studies investigating climate variability and health dominate the literature, leaving predictive and prospective studies related to climate change open to be explored. However, both prospective and retrospective studies need high-quality data. Working groups at the Goa workshop were able to identify existing and relevant long-term data sets that can be used for environmental epidemiological analysis. For example, both the Indian Institute of Tropical Meteorology ( IITM 2010 ) and the India Meteorological Department (2010) have useful meteorological data with varying degrees of access. Additional government surveys such as the Census of India and the National Family Health Survey, India provide important information on social and economic variables. In some cases, individual investigators have accessed government hospital data sets and have daily all-cause mortality, albeit over a limited geography. The same goes for air pollution data, such as data on particulate matter (PM), which have been accessed at certain locations of interest, such as Chennai ( Ghosh et al. 2010 ). The use of exposure and emission models can help to fill in where air pollution data are missing; however, consistent monitoring of PM, ozone, and nitrogen oxides over a greater geographic area is needed. In cases where the data already exist, more work is needed to identify and access this type of long-term data, creating uniform repositories. In cases where it does not exist, surveillance and monitoring of relevant variables will be critical to the success of future prospective climate and health research endeavors. Furthermore, regional climate models for India such as PRECIS (Providing Regional Climates for Impacts Studies) developed at the IITM must be integrated with health data if we are going to transition away from surveying the health effects associated with climate variability to predicting the effects of climate change.
Changes to the current information infrastructure needed for this effort will depend on new or enhanced collaborations across multiple disciplines and among diverse institutions. Given the region-specific nature of the relationship between climate variability and health, further research is required throughout India. Satellite and geospatial technology may provide new insights regarding the geographic distribution of risk and disease. Integration of social, demographic, and land cover data with health data will aid in describing a holistic health scenario, which will help identify sustainable health solutions. These research needs and methodological limitations are relevant to many low- and middle-income countries. India, with its current health infrastructure and large population, can serve as an important natural laboratory for developing relevant strategies for promoting and managing climate health research in the developing world.
Recommendations
As a result of the Goa workshop and subsequent discussions, the following recommendations to advance research relevant to climate change and human health were proposed.
Environmental monitoring and surveillance
There is a great need to improve environmental monitoring and surveillance systems in low- and middle-income countries such as India. New research initiatives should focus on collecting high-quality, long-term data on climate-related health outcomes with the dual purpose of understanding current climate–health associations and predicting future scenarios. Health outcomes of interest, for which such data should be collected, include total morbidity and mortality and noncommunicable diseases such as cardiovascular, respiratory, and circulatory diseases and asthma, as well as infectious diseases such as cholera, malaria, tuberculosis, typhoid, hepatitis, dysentery, tick-borne encephalitis, and other vector-borne and waterborne diseases. Such monitoring also requires the collection of appropriate climatic (e.g., temperature and precipitation) and nonclimatic data (e.g., ozone). Surveillance of extreme weather conditions and risk indicators such as mosquito abundance or pathogen load is also necessary. Such data gathering should occur in conjunction with already existing public health programs and health centers. Where the necessary public health infrastructure does not exist, the anticipated risks associated with climate change should motivate international action to build such infrastructure. The collection of such diverse data necessitates the creation of linkable and documented repositories for meteorological, air pollution, and health data. Such a virtual network, or clearinghouse, will help researchers as well as practitioners as they work toward defining climate–health associations and designing effective interventions. Such monitoring provides the information and feedback necessary to take action in response to the anticipated changes in climate and burden on the public health infrastructure.
Geospatial technology
Geographic information systems and spatial analysis must be further developed; they are very useful tools when conducting vulnerability assessments, assessing environmental exposures, prioritizing research, and disseminating findings to decision makers and the public alike ( Jerrett et al. 2010 ). Remote sensing and environmental monitoring are particularly useful to catalog variables such as air pollution and heat exposure. Social data from census and surveys, which can be layered with the exposure data using geographic information systems, provide information on sensitivity and adaptive capacity, at both individual and community levels. Data on land use and land cover can provide additional information on relevant environmental factors that influence risk and vulnerability.
Such a spatial information infrastructure provides the necessary data-integration framework to combine information on human–environment interactions from a variety of sources. Vulnerability assessments can be conducted spatially and temporally through integration of such social and environmental data. Risk maps can incorporate social and ecological risk factors in an attempt to characterize the existing spatial heterogeneity. This is a very effective tool when predicting prevalence, targeting resource distribution, and designing control programs for different infectious diseases such as malaria ( Ageep et al. 2009 ; Haque et al. 2010 ; Reid et al. 2010 ; Tonnang et al. 2010 ). An example of such work, which grew out of the Goa workshop, will focus on the effect of socioeconomic status on the association between climate and malaria.
Human and technical capacity
For these new surveillance methods and analytical techniques to be effective, countries like India will need to enhance their human and technical capacity for risk communication. This could take the form of public education on climate change and associated health impacts to enhance awareness and to influence lifestyle, behavior, and individual choices to protect and improve health. Such health promotion materials could manifest as low-tech flyers and advertisements as well as more high-tech materials including web-based and mobile-phone–based alerts. On the other end of the spectrum, developing capacity could take on a more holistic approach, such as region- and city-specific climate action plans and early warning system for heat stress events, droughts, hurricanes, and floods.
Studies of climate variability and human health indicate a great deal of heterogeneity in the reported associations. This heterogeneity is partially due to differences in study design, but climatic and socioeconomic differences that vary by location also influence the burden of disease. It is not clear whether results from one region can be extrapolated to others. Therefore, it is important to develop a comprehensive catalog of climate change and associated health outcomes across the range of environments and populations likely to be affected. A better understanding of the effects of climate change on health in India will be best achieved through studies specific to climates and populations in India.
In 2008 India developed the National Action Plan on Climate Change, promising further enhancement of ecological sustainability as part of India’s development path, signaling their involvement in the international discussion on climate change. Countries like India have a tremendous opportunity to guide our future trajectory regarding sustainable development and adaptation to climate change, but it will take the combined effort of policy makers and scientists from around the world to address the complex challenges associated with climate change and human health.
In conclusion, innovative, multidisciplinary investigations using environmental epidemiologic methods to elucidate health risks posed by climate variability—and subsequent climate change—in regions such as India are possible. However, such work will require expanded partnerships among researchers, governments, and communities to develop a cobenefit strategy that addresses public health challenges and risks associated with climate change. Adoption and implementation of these research initiatives will provide the necessary tools and infrastructure to pose interesting scientific questions and design effective solutions to the complex issues imposed by climate change.
We thank all of the participants who contributed to the success of the 2009 Joint Indo-U.S. Workshop on Climate Change and Health, particularly K. Knowlton and A. Jaiswal from the Natural Resources Defense Council; S. Nair and colleagues at the Energy and Resources Institute; D. Sur from the National Institute of Cholera and Enteric Diseases; partners from across the Indian Institutes of Technology, including S. Dash and M. Khare; those at the Indian Institute of Tropical Meteorology, including G. Beig and K. Kumar; and S. Reddy and associates from the Public Health Foundation of India.
This work is based on the 2009 workshop in Goa, India, which was principally supported by the University of Michigan Center for Global Health, the U.S. Centers for Disease Control and Prevention, and the Indian Council for Medical Research. K.F.B. was supported by a University of Michigan Graham Environmental Sustainability Institute doctoral fellowship.
The findings, conclusions, and recommendations in this report are those of the authors and do not necessarily represent the official position of the Center for Global Health, the Centers for Disease Control and Prevention, or the Indian Council of Medical Research.
- Agarwal R, Jayaraman G, Anand S, Marimuthu P. Assessing respiratory morbidity through pollution status and meteorological conditions for Delhi. Environ Monit Assess. 2006; 114 (1–3):489–504. [ PubMed ] [ Google Scholar ]
- Ageep TB, Cox J, Hassan MM, Knols BGJ, Benedict MQ, Malcolm CA, et al. Spatial and temporal distribution of the malaria mosquito Anopheles arabiensis in northern Sudan: influence of environmental factors and implications for vector control. Malar J. 2009; 8 :123. doi: 10.1186/1475-2875-8-123. [Online 7 June 2009] [ PMC free article ] [ PubMed ] [ CrossRef ] [ Google Scholar ]
- Agrawal A. Local institutions and adaptation to climate change. In: Mearns R, Norton A, editors. Social Dimensions of Climate Change: Equity and Vulnerability in a Warming World. Washington, DC: World Bank; 2009. pp. 173–198. [ Google Scholar ]
- Akhtar R, McMichael AJ. Rainfall and malaria outbreaks in western Rajasthan. Lancet. 1996; 348 :1457–1458. [ PubMed ] [ Google Scholar ]
- Batterman S, Eisenberg J, Hardin R, Kruk ME, Lemos MC, Michalak AM, et al. Sustainable control of water-related infectious diseases: a review and proposal for interdisciplinary health-based systems research. Environ Health Perspect. 2009; 117 :1023–1032. [ PMC free article ] [ PubMed ] [ Google Scholar ]
- Bhattacharya S, Sharma C, Dhiman RC, Mitra AP. Climate change and malaria in India. Curr Sci. 2006; 90 :369–375. [ Google Scholar ]
- Bouma MJ, van der Kaay HJ. Epidemic malaria in India and the El Nino Southern Oscillation. Lancet. 1994; 344 :1638–1639. [ PubMed ] [ Google Scholar ]
- Burke J. Hundreds Die in Indian Heatwave. Guardian 30 May. 2010. [[accessed 18 November 2010]]. Available: http://www.guardian.co.uk/world/2010/may/30/india-heatwave-deaths
- Dash AP, Valecha N, Anvikar AR, Kumar A. Malaria in India: challenges and opportunities. J Biosci. 2008; 33 :583–592. [ PubMed ] [ Google Scholar ]
- Devi NP, Jauhari RK. Climatic variables and malaria incidence in Dehradun, Uttaranchal, India. J Vector Borne Dis. 2006; 43 :21–28. [ PubMed ] [ Google Scholar ]
- Dhiman RC, Pahwa S, Dash AP. Climate change and Malaria in India: interplay between temperature and mosquitoes. Regional Health Forum. 2008; 12 :27–31. [ Google Scholar ]
- Dhiman RC, Pahwa S, Dhillon GPS, Dash AP. Climate change and threat of vector-borne disease in India: are we prepared? Parasitol Res. 2010; 106 :763–773. [ PubMed ] [ Google Scholar ]
- Dhingra N, Jha P, Sharma VP, Cohen AA, Jotkar RM, Rodriguez PS, et al. Adult and child malaria mortality in India: a nationally representative mortality survey. Lancet. 2010; 376 :1768–1774. [ PMC free article ] [ PubMed ] [ Google Scholar ]
- Doherty RM, Heal MR, Wilkinson P, Pattenden S, Vieno M, Armstrong B, et al. Current and future climate- and air pollution-mediated impacts on human health. Environ Health (suppl 1) 2009:S8. doi: 10.1186/1476-069X-8-S1-S8. [Online 21 December 2009] [ PMC free article ] [ PubMed ] [ CrossRef ] [ Google Scholar ]
- Ebi KL, Kovats RS, Menne B. An approach for assessing human health vulnerability and public health interventions to adapt to climate change. Environ Health Perspect. 2006; 114 :1930–1934. [ PMC free article ] [ PubMed ] [ Google Scholar ]
- Ebi KL, Paulson JA. Climate change and child health in the United States. Curr Probl Pediatr Adolesc Health Care. 2010; 40 :2–18. [ PubMed ] [ Google Scholar ]
- Ebi KL, Semenza JC. Community-based adaptation to the health impacts of climate change. Am J Prev Med. 2008:35501–35507. [ PubMed ] [ Google Scholar ]
- Ghosh S, Johnson P, Ravinder S, Chakraborty M, Mittal M, Balakrishnan K. Development and application of spatially disaggregated exposure series in time-series analyses of air pollution-related health effects in Chennai, India. Epidemiology. 2010; 22 :S81–S82. [ Google Scholar ]
- Goswami BN, Venugopal V, Sengupta D, Madhusoodanan MS, Xavier PK. Increasing trend of extreme rain events over India in a warming environment. Science. 2006; 314 (5804):1442–1445. [ PubMed ] [ Google Scholar ]
- Haines A, Kovats RS, Campbell-Lendrum D, Corvalan C. Climate change and human health: impacts, vulnerability, and public health. Public Health. 2006; 120 :585–596. [ PubMed ] [ Google Scholar ]
- Hajat S, Armstrong BG, Gouvia N, Wilkinson P. Mortality displacement of heat-related deaths: a comparison of Delhi, São Paulo, and London. Epidemiology. 2005; 16 :613–620. [ PubMed ] [ Google Scholar ]
- Haque U, Magalhães RJS, Reid HL, Clements ACA, Ahmed SM, Islam A, et al. Spatial prediction of malaria prevalence in an endemic area of Bangladesh. Malar J. 2010; 9 :120. doi: 10.1186/1475-2875-9-120. [Online 9 May 2010] [ PMC free article ] [ PubMed ] [ CrossRef ] [ Google Scholar ]
- India Meteorological Department. India Meteorological Department, Pune. 2010. [[accessed 10 November 2010]]. Available: http://www.imdpune.gov.in/
- Indian Institute of Tropical Meteorology. IITM: A World Centre of Excellence in Basic Research on the Ocean–Atmosphere Climate System Required for Improvement of Weather and Climate Forecasts. 2010. [[accessed 10 November 2010]]. Available: http://www.tropmet.res.in
- Pachauri RK, Reisinger A, editors. Intergovernmental Panel on Climate Change; Core Writing Team. Climate Change 2007: Synthesis Report. Contribution of Working Groups I, II and III to the Fourth Assessment Report of the Intergovernmental Panel on Climate Change. Geneva: Intergovernmental Panel on Climate Change; 2007. [ Google Scholar ]
- International Institute for Population Sciences and Macro International. National Family Health Survey (NFHS-3), 2005–06: India. Vol. 1. Mumbai: International Institute for Population Sciences and Macro International; 2007. [ Google Scholar ]
- Jerrett M, Gale S, Kontgis C. Spatial modeling in environmental and public health. Int J Environ Res Public Health. 2010; 7 :1302–1329. [ PMC free article ] [ PubMed ] [ Google Scholar ]
- Karar K, Gupta AK, Kumar A, Biswas AK. Seasonal variations of PM 10 and TSP in residential and industrial sites in an urban area of Kolkata, India. Environ Monit Assess. 2006; 118 :369–381. [ PubMed ] [ Google Scholar ]
- Kumar A, Valecha N, Jain T, Dash AP. Burden of malaria in India: retrospective and prospective view. Am J Trop Med Hyg. 2007; 77 (6 suppl):69–78. [ PubMed ] [ Google Scholar ]
- Laneri K, Bhadra A, Ionides EL, Bouma M, Dhiman RC, Yadav RS, et al. Forcing versus feedback: epidemic malaria and monsoon rains in northwest India. PLoS Comput Biol. 2010; 6 (9) doi: 10.1371/journal.pcbi.1000898. [Online 2 September 2010] [ PMC free article ] [ PubMed ] [ CrossRef ] [ Google Scholar ]
- Mandal K. Drinking Water Supply vis-a-vis Technological Interventions for Social Empowerment of Rural India. 2008. [[accessed 23 May 2010]]. Available: http://www.nistads.res.in/indiasnt2008/t6rural/t6rur7.htm
- McMichael A. Climate change. In: Ezzati M, Lopez A, Rodgers A, Murray C, editors. Comparative Quantification of Health Risks: Global and Regional Burden of Disease due to Selected Major Risk Factors. Vol. 2. Geneva: World Health Organization; 2004. pp. 1543–1649. [ Google Scholar ]
- McMichael AJ, Campbell-Lendrum DH, Corvalán CF, Ebi KL, Githeko A, Scheraga JD, et al. Climate Change and Human Health—Risks and Responses. Geneva: World Health Organization; 2003. [ Google Scholar ]
- McMichael AJ, Wilkinson P, Kovats RS, Pattenden S, Hajat S, Armstrong B, et al. International study of temperature, heat and urban mortality: the “ISOTHURM” project. Int J Epidemiol. 2008; 37 :1121–1131. [ PubMed ] [ Google Scholar ]
- Narain JP. Malaria in the South-East Asia region: myth and the reality. Indian J Med Res. 2008; 128 :1–3. [ PubMed ] [ Google Scholar ]
- O’Neill MS, Ebi KL. Temperature extremes and health: impacts of climate variability and change in the United States. J Occup Environ Med. 2009; 51 :13–25. [ PubMed ] [ Google Scholar ]
- Pande JN, Bhatta N, Biswas D, Pandey RM, Ahluwalia G, Siddaramaiah NH. Outdoor air pollution and emergency room visits at a hospital in Delhi. Indian J Chest Dis Allied Sci. 2002; 44 :13–19. [ PubMed ] [ Google Scholar ]
- Pascual M, Dobson AP, Bouma MJ. Underestimating malaria risk under variable temperatures. Proc Natl Acad Sci USA. 2009; 106 :13645–13646. [ PMC free article ] [ PubMed ] [ Google Scholar ]
- Patz JA, Campbell-Lendrum D, Holloway T, Foley JA. Impact of regional climate change on human health. Nature. 2005; 17 :310–317. [ PubMed ] [ Google Scholar ]
- Patz JA, Gibbs HK, Foley JA, Rogers JV, Smith KR. Climate change and global health: quantifying a growing ethical crisis. EcoHealth. 2007; 4 :397–405. [ Google Scholar ]
- Patz JA, Hulme M, Rosenzweig C, Mitchell TD, Goldberg RA, Githeko AK, et al. Climate change: regional warming and malaria resurgence. Nature. 2002; 420 :627–628. [ PubMed ] [ Google Scholar ]
- Patz JA, Olson SH. Climate change and health: global to local influences on disease risk. Ann Trop Med Parasitol. 2006; 100 :535–549. [ PubMed ] [ Google Scholar ]
- Pulikesi M, Baskaralingam P, Elango D, Rayudu VN, Ramamurthi V, Sivanesan S. Air quality monitoring in Chennai, India, in the summer of 2005. J Hazard Mater. 2006; 136 :589–596. [ PubMed ] [ Google Scholar ]
- Reid H, Haque U, Clements ACA, Tatem AJ, Vallely A, Ahmed SM, et al. Mapping malaria risk in Bangladesh using Bayesian geostatistical models. Am J Trop Med Hyg. 2010; 83 :861–867. [ PMC free article ] [ PubMed ] [ Google Scholar ]
- Sattenspiel L. Tropical environments, human activities, and the transmission of infectious diseases. Am J Phys Anthropol. 2000; 31 :3–31. [ PubMed ] [ Google Scholar ]
- Shope R. Global climate change and infectious diseases. Environ Health Perspect. 1991; 96 :171–174. [ PMC free article ] [ PubMed ] [ Google Scholar ]
- Singh MR, Upadhyay V, Mittal AK. Addressing sustainability in benchmarking framework for Indian urban water utilities. J Infrastr Systems. 2010; 16 :81–92. [ Google Scholar ]
- Singh N, Chand SK, Mishra AK, Nagpal AC. Migration malaria associated with forest economy in central India. Curr Sci. 2004; 87 :1696–1699. [ Google Scholar ]
- Smith KR. National burden of disease in India from indoor air pollution. Proc Natl Acad Sci USA. 2000; 97 :13286–13293. [ PMC free article ] [ PubMed ] [ Google Scholar ]
- Tonnang HEZ, Kangalawe RYM, Yanda PZ. Predicting and mapping malaria under climate change scenarios: the potential redistribution of malaria vectors in Africa. Malar J. 2010; 9 :111. doi: 10.1186/1475-2875-9-111. [Online 23 April 2010] [ PMC free article ] [ PubMed ] [ CrossRef ] [ Google Scholar ]
- WHO (World Health Organization) World Health Report 200—Changing History. Geneva: WHO; 2004. [ Google Scholar ]
- WHO. (World Health Organization) World Malaria Report 2008. Geneva: WHO; 2008. [ Google Scholar ]
- WHO and UNICEF (World Health Organization and United Nations Children’s Fund) Water Sanitation and Health (WSH). Global Water Supply and Sanitation Assessment 2000 Report. Geneva: WHO; 2000. [ Google Scholar ]
- Wiley LF, Gostin LO. The international response to climate change: an agenda for global health. JAMA. 2009; 302 :1218–1220. [ PubMed ] [ Google Scholar ]
- Zuckerman JN, Rombo L, Fisch A. The true burden and risk of cholera: implications for prevention and control. Lancet Infect Dis. 2007; 7 :521–530. [ PubMed ] [ Google Scholar ]
Yale Program on Climate Change Communication
- About YPCCC
- Yale Climate Connections
- Student Employment
- For The Media
- Past Events
- YPCCC in the News
- Climate Change in the American Mind (CCAM)
Publications
- Climate Opinion Maps
- Climate Opinion Factsheets
- Six Americas Super Short Survey (SASSY)
- Resources for Educators
- All Tools & Interactives
- Partner with YPCCC
Home / Global Warming’s Six Indias
Report · May 21, 2013
Global warming’s six indias, filed under: beliefs & attitudes, report summary.
A new national study in India finds six distinct groups within the Indian public that respond to the issue of climate change in very different ways. These “Six Indias” include:
- The Informed (19%)
- The Experienced (24%)
- The Undecided (15%)
- The Unconcerned (15%)
- The Indifferent (11%)
- The Disengaged (16%)
An earlier report provides topline results broken down by sociodemographics: Climate Change in the Indian Mind
Download Full Report
PDF Document
International Attitudes & Behavior
Related Work
Report · mar 26, 2024, international public opinion on climate ..., report · mar 7, 2024, climate change in the irish mind, 2023, report · nov 14, 2023, report · oct 3, 2023, climate change in the indonesian mind, climate note · aug 1, 2023, global warming’s six audiences: a cros..., report · may 4, 2023, global warming’s four indias, 2022..., report · mar 7, 2023, climate change’s four irelands: an..., report · oct 19, 2022, climate change in the indian mind, 2022, report · jun 29, 2022, report · apr 6, 2022, climate change in the brazilian mind, climate note · feb 28, 2022, environmental beliefs among business stu..., report · dec 14, 2021, climate change in the irish mind, report · oct 26, 2021, public support for international climate..., report · jun 28, 2021, report · apr 19, 2021, climate note · may 23, 2017, media use and public perceptions of glob..., climate note · may 8, 2017, majorities of americans in every state s..., climate note · nov 25, 2015, americans support an international clima..., peer-reviewed article · jul 27, 2015, climate change awareness and concern in ..., peer-reviewed article · mar 4, 2014, shifting discourses of climate change in..., peer-reviewed article · dec 15, 2012, global perceptions of local temperature ..., report · nov 28, 2012, public climate change awareness and clim..., report · aug 26, 2012, climate change in the indian mind, peer-reviewed article · mar 15, 2012, rio+20: an endangered species, subscribe to our mailing list.
Please select all the ways you would like to hear from Yale Program on Climate Change Communication:
You can unsubscribe at any time by clicking the link in the footer of our emails. For information about our privacy practices, please visit our website.
We use Mailchimp as our marketing platform. By clicking below to subscribe, you acknowledge that your information will be transferred to Mailchimp for processing. Learn more about Mailchimp's privacy practices here.
Advertisement
Supported by
Hot Oceans Worsened Dubai’s Dramatic Flooding, Scientists Say
An international team of researchers found that heavy rains had intensified in the region, though they couldn’t say for sure how much climate change was responsible.
- Share full article

By Raymond Zhong
Scenes of flood-ravaged neighborhoods in one of the planet’s driest regions stunned the world this month. Heavy rains in the United Arab Emirates and Oman submerged cars, clogged highways and killed at least 21 people. Flights out of Dubai’s airport, a major global hub, were severely disrupted.
The downpours weren’t a total surprise — forecasters had anticipated the storms several days earlier and issued warnings. But they were certainly unusual.
Here’s what to know.
Heavy rain there is rare, but not unheard-of.
On average, the Arabian Peninsula receives a scant few inches of rain a year, although scientists have found that a sizable chunk of that precipitation falls in infrequent but severe bursts, not as periodic showers. These rains often come during El Niño conditions like the ones the world is experiencing now.
U.A.E. officials said the 24-hour rain total on April 16 was the country’s largest since records there began in 1949 . And parts of the nation had already experienced an earlier round of thunderstorms in March.
Oman, with its coastline on the Arabian Sea, is also vulnerable to tropical cyclones. Past storms there have brought torrential rain, powerful winds and mudslides, causing extensive damage.
Global warming is projected to intensify downpours.
Stronger storms are a key consequence of human-caused global warming. As the atmosphere gets hotter, it can hold more moisture, which can eventually make its way down to the earth as rain or snow.
But that doesn’t mean rainfall patterns are changing in precisely the same way across every part of the globe.
In their latest assessment of climate research , scientists convened by the United Nations found there wasn’t enough data to have firm conclusions about rainfall trends in the Arabian Peninsula and how climate change was affecting them. The researchers said, however, that if global warming were to be allowed to continue worsening in the coming decades, extreme downpours in the region would quite likely become more intense and more frequent.
Hot oceans are a big factor.
An international team of scientists has made a first attempt at estimating the extent to which climate change may have contributed to April’s storms. The researchers didn’t manage to pin down the connection precisely, though in their analysis, they did highlight one known driver of heavy rain in the region: above-normal ocean temperatures.
Large parts of the Indian, Pacific and Atlantic Oceans have been hotter than usual recently, in part because of El Niño and other natural weather cycles, and in part because of human-induced warming .
When looking only at El Niño years, the scientists estimated that storm events as infrequent as this month’s delivered 10 percent to 40 percent more rain to the region than they would in a world that hadn’t been warmed by human activities. They cautioned, however, that these estimates were highly uncertain.
“Rainfall, in general, is getting more extreme,” said Mansour Almazroui, a climate scientist at King Abdulaziz University in Jeddah, Saudi Arabia, and one of the researchers who contributed to the analysis.
The analysis was conducted by scientists affiliated with World Weather Attribution, a research collaboration that studies extreme weather events shortly after they occur. Their findings about this month’s rains haven’t yet been peer reviewed, but are based on standardized methods .
The role of cloud seeding isn’t clear.
The U.A.E. has for decades worked to increase rainfall and boost water supplies by seeding clouds. Essentially, this involves shooting particles into clouds to encourage the moisture to gather into larger, heavier droplets, ones that are more likely to fall as rain or snow.
Cloud seeding and other rain-enhancement methods have been tried around the world, including in Australia, China, India, Israel, South Africa and the United States. Studies have found that these operations can, at best, affect precipitation modestly — enough to turn a downpour into a bigger downpour, but probably not a drizzle into a deluge.
Still, experts said pinning down how much seeding might have contributed to this month’s storms would require detailed study.
“In general, it is quite a challenge to assess the impact of seeding,” said Luca Delle Monache, a climate scientist at the Scripps Institution of Oceanography in La Jolla, Calif. Dr. Delle Monache has been leading efforts to use artificial intelligence to improve the U.A.E.’s rain-enhancement program.
An official with the U.A.E.’s National Center of Meteorology, Omar Al Yazeedi, told news outlets that the agency didn’t conduct any seeding during the latest storms. His statements didn’t make clear, however, whether that was also true in the hours or days before.
Mr. Al Yazeedi didn’t respond to emailed questions from The New York Times, and Adel Kamal, a spokesman for the center, didn’t have further comment.
Cities in dry places just aren’t designed for floods.
Wherever it happens, flooding isn’t just a matter of how much rain comes down. It’s also about what happens to all that water once it’s on the ground — most critically, in the places people live.
Cities in arid regions often aren’t designed to drain very effectively. In these areas, paved surfaces block rain from seeping into the earth below, forcing it into drainage systems that can easily become overwhelmed.
One recent study of Sharjah , the capital of the third-largest emirate in the U.A.E., found that the city’s rapid growth over the past half-century had made it vulnerable to flooding at far lower levels of rain than before.
Omnia Al Desoukie contributed reporting.
Raymond Zhong reports on climate and environmental issues for The Times. More about Raymond Zhong
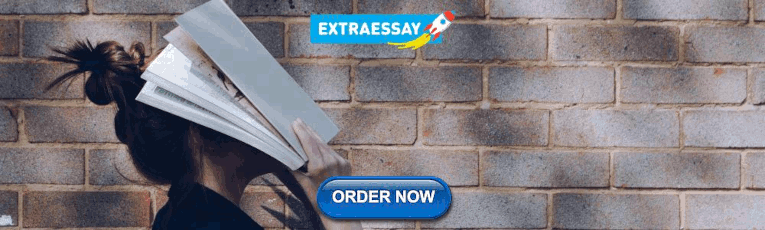
COMMENTS
According to a study by the International Energy Agency (IEA), India emitted 2,299 million tonnes of carbon dioxide (CO 2) in 2018, a rise of 4.8% over the previous year.". Unfortunately, India's future potential emissions are not yet aligned with the Paris Agreement goals. India's NDCs currently correspond to temperature increases above ...
This is where climate adaptation becomes critical. While mitigation involves cutting the pace of global emissions and slowing down warming, adaptation is essential to save lives and livelihoods in the short to medium term. Adaptation also helps in building long-term resilience to the impacts of climate change.
India, one of the most disaster-prone countries in the world, has suffered severe economic losses as well as life losses as per the World Focus report.1 More than 80% of its land and more than 50 million of its people are affected by weather disasters. Disaster mitigation necessitates reliable future predictions, which need focused climate change research. From the climate change perspective ...
Some researchers say similar conditions may have loosed floods that killed 3,000 people in Pakistan and northern India in the summer of 2010. Several recent research papers say that global warming may have set up Kednarath for the disaster, by transforming regional weather patterns and eroding Chorabari Glacier.
This is the need of the hour.". From heat waves devastating crop yields to torrential rains causing flooding that submerges entire communities, India is experiencing some of the most extreme impacts of the climate crisis. While India comprises a little over 17% of the world's population, it produces just about 7% of global emissions.
Our analyses find significant warming in India in most seasons and regions, which is consistent with a previous study for the period 1901-2003 by Pal and Al-Tabba 11. Surface temperature of ...
Climate change is a global concern of the current century. Its rapid escalation and ever-increasing intensity have been felt worldwide, leading to dramatic impacts globally. The aftermath of climate change in India has brought about a profound transformation in India's environmental, socio-economic, and urban landscapes. In 2019, India ranked seventh, among the most affected countries by ...
Human-induced climate change made the deadly heatwave that gripped India and Pakistan in March and April 30 times more likely, according to a rapid analysis of the event. Temperatures began rising ...
A. Sahai. Published 4 November 1998. Environmental Science, Geography. Theoretical and Applied Climatology. Summary A brief account of various causes of climate change in recent decades and climate change trends in the Indian region is presented. It is of great importance to determine the influence of human activities on the likely climate ...
Climate-sensitive diseases are among the largest global killers. Diarrhoea, malaria and protein-energy malnutrition alone caused more than 3.3 million deaths globally in 2002, with 29% of these deaths occurring in the Region of Africa. No, we are not even saying that India is the only country taking the entire brunt of global warming.
It is mentioned in the literature that there is either a cooling tendency or cessation of warming after the late 1950s at most of the Indian industrial cities. A case study of Nagpur, a centrally located city in India, is done to understand and the possible cause of cooling. ... A. Climate Change: A Case Study Over India. Theor Appl Climatol 61 ...
It should come as little surprise, therefore, that India has been at the forefront of driving global action on climate change. It has used indigenous technology to optimize its resources and promoted green energy to reduce carbon emissions; Prime Minister Narendra Modi's announcement to establish a National Hydrogen Mission is noteworthy.
In this study, we examined climate change risk perceptions among the Indian public. When asked for the first word or phrase that comes to mind when thinking about global warming, the single most frequent response among Indians was "don't know" or "can't say" (25%), reflecting a critical difference in issue awareness compared to, for ...
In their most recent NDC India estimated that at least USD 2.5 trillion (at 2014-15 prices) will be required for meeting its climate change actions between 2016 and 2030. And the International ...
Murali Krishnan in New Delhi. 07/11/2023. As torrential rains cause flash floods and landslides in India, the country grapples with an environmental crisis. The heavy rain comes after an ...
In a second study based in Chennai, India, Ghosh et al. (2010) concluded that short-term exposure to particulate matter ≤ 10 μm in aerodynamic diameter (PM 10) resulted in an estimated risk ratio of 1.0044 (95% CI, 1.002-1.007) per a 10 μg/m 3 increase in daily average concentrations; this risk estimate is comparable to similar estimates ...
7) Way Forward. To limit ourselves to 1.5°C, global net anthropogenic CO2 emissions should reduce by about 45 per cent from 2010 levels by 2030, and should reach net-zero around 2050. Use of coal should reduce steeply and its share in electricity mix should be reduced to close to 0 per cent by 2050. To limit global warming, countries will have ...
There has been a particularly alarming effect of global warming on the climate of India. India is already a disaster prone area, with the statistics of 27 out of 35 states being disaster prone, with most disasters being water related. The process of global warming has led to an increase in the frequency and intensity of these climatic disasters.
The results suggest that efforts to increase the quantity and quality of Indian media coverage of global warming, including stories connecting the issue to changes in local weather patterns and extremes, is likely an effective communication strategy in India, the world's second-most populous country, where approximately 40% of adults (or ...
The residential floor area per person in Delhi is 3.31 square metres, compared to 21.85 square meters in Beijing. The mean diurnal range of Delhi's ambient temperature has also been steadily decreasing due to urban heat island effects. Under low wind conditions which commonly prevail in Delhi, external polluted air can be drawn into the city.
Report Summary. A new national study in India finds six distinct groups within the Indian public that respond to the issue of climate change in very different ways. These "Six Indias" include: The Informed (19%) The Experienced (24%) The Undecided (15%) The Unconcerned (15%) The Indifferent (11%)
Global warming is projected to intensify downpours. ... China, India, Israel, South Africa and the United States. ... Studies have found that these operations can, at best, affect precipitation ...
In our Global Offshore Wind Update Report released before COP28, we referenced how the International Renewable Energy Agency (IRENA) forecasted that the world needs a threefold increase in total renewable generation by 2030 if global warming is to remain below 1.5°C.