REVIEW article
Recent progress in lyme disease and remaining challenges.
- 1 Icahn School of Medicine at Mount Sinai, New York, NY, United States
- 2 Department of Biochemistry, Fralin Life Sciences Institute, Virginia Tech, Blacksburg, VA, United States
- 3 Lyme Disease Biobank, Portland, OR, United States
- 4 Tulane University Health Sciences, New Orleans, LA, United States
- 5 Institute for Systems Biology, Seattle, WA, United States
- 6 State Key Laboratory for the Diagnosis and Treatment of Infectious Diseases, The First Affiliated Hospital, Zhejiang University School of Medicine, Hangzhou, China
- 7 Division of Rheumatology, Department of Medicine, Lyme Disease Research Center, Johns Hopkins University School of Medicine, Baltimore, MD, United States
- 8 Cary Institute of Ecosystem Studies, Millbrook, NY, United States
- 9 Department of Microbiology and Immunology, Virginia Commonwealth University Medical Center, Richmond, VA, United States
- 10 Bard College, Annandale, NY, United States
- 11 Department of Biology, Northeastern University, Boston, MA, United States
- 12 Yale University School of Medicine, New Haven, CT, United States
- 13 Department of Clinical Sciences, Comparative Medicine Institute, College of Veterinary Medicine, North Carolina State University, Raleigh, NC, United States
- 14 Department of Microbiology and Immunology, Indiana University School of Medicine, Indianapolis, IN, United States
- 15 Steven & Alexandra Cohen Foundation, Stamford, CT, United States
- 16 University of California, Los Angeles, Los Angeles, CA, United States
- 17 Department of Psychiatry and Behavioral Sciences, Johns Hopkins University School of Medicine, Baltimore, MD, United States
- 18 Center for Immunology and Infectious Diseases and the Department of Pathology, Microbiology & Immunology, School of Veterinary Medicine, University of California, Davis, Davis, CA, United States
- 19 Columbia University Irving Medical Center, New York, NY, United States
Lyme disease (also known as Lyme borreliosis) is the most common vector-borne disease in the United States with an estimated 476,000 cases per year. While historically, the long-term impact of Lyme disease on patients has been controversial, mounting evidence supports the idea that a substantial number of patients experience persistent symptoms following treatment. The research community has largely lacked the necessary funding to properly advance the scientific and clinical understanding of the disease, or to develop and evaluate innovative approaches for prevention, diagnosis, and treatment. Given the many outstanding questions raised into the diagnosis, clinical presentation and treatment of Lyme disease, and the underlying molecular mechanisms that trigger persistent disease, there is an urgent need for more support. This review article summarizes progress over the past 5 years in our understanding of Lyme and tick-borne diseases in the United States and highlights remaining challenges.
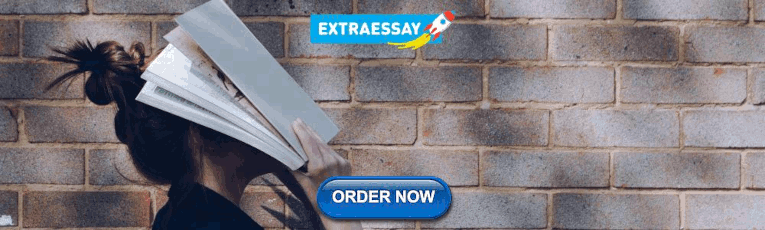
Introduction
According to the Center for Disease Control and Prevention (CDC), the number of vector-borne diseases reported to the National Notifiable Diseases Surveillance System (NNDSS) between 2004 and 2016 reached a total of 642,602 cases. Of these, tick-borne diseases (TBDs) accounted for 77% (491,671 cases) of reported cases with the total number of cases doubling in 13 years. The pace of emergence of new tick-borne disease cases increased not only for Lyme disease (LD), the most predominant TBD with 82% of cases, but also for spotted fever rickettsiosis, babesiosis, anaplasmosis, and Powassan disease ( 1 ). In this review, we highlight the major scientific advances made primarily in the field of LD research in the United States (USA).
LD, also known as Lyme borreliosis, is a growing health problem in the USA. LD is caused by pathogenic species in the Borreliella genus (for the relationship with the Borrelia genus, see section Genomic Insights From Borreliaceae Lineages). These spirochetal bacteria are transmitted from vertebrate reservoirs to human hosts through bites from infected Ixodes spp . ticks. Borreliella burgdorferi (B. burgdorferi , hereafter Bb) is the most common agent of LD in the USA ( 1 ). The CDC recently estimated ~476,000 clinician diagnosed cases of LD every year in the USA based on insurance claims data from 2010 to 2018 ( 2 ), a significant increase from their previous estimate of ~329,000 annual cases using similar methods to generate data from 2005 to 2010 ( 3 ). If untreated, infection with Bb can lead to health problems affecting the skin, joints, nervous system, or less commonly, the heart ( 4 ). While most individuals return to health following antibiotic treatment for LD, others go on to experience chronic health problems that can last months to years. One well-defined clinical subgroup of LD patients who experience ongoing symptoms following treatment is Post-treatment Lyme disease (PTLD) (see section 2.3.2 PTLD). Medical costs related to LD and PTLD are estimated to be between $712M−1.3B each year in the USA ( 5 ). The causes of PTLD are not yet well-understood but are an active area of research due to their critical importance to advancing therapy development and effective treatment for this patient population. The two most salient hypotheses for etiology of PTLD include persistence of infection or antigenic debris, persistence of inappropriate immune activation and inflammation, or some combination of these (see section Pathogenesis below). The research community has largely lacked the necessary funding to properly advance scientific and clinical understanding of LD and its sequelae, and to develop and evaluate new approaches for prevention, diagnosis, and treatment. The annual NIH investment in LD research so far has been small compared to many other infectious diseases (see Table 1 ) ( 6 ).
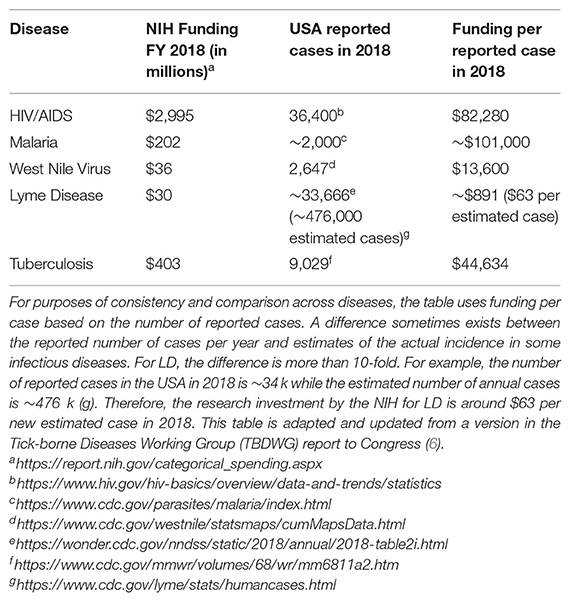
Table 1 . NIH support for LD research is currently low compared to other infectious diseases.
Considering the rapid growth in prevalence of LD and the risk for significant long-term health consequences of those infected ( 7 ), a multifaceted effort is needed to create better prevention practices, diagnostics, and treatments, along with advancing basic science about ticks, tick-borne pathogens, and the pathophysiology of LD. In this review, we summarize key advances in each of these areas over the past 5 years and identify challenges and opportunities for the field. We aim to highlight many important studies, but due to space constraints, we are not able to discuss all relevant publications. Where available, we also reference more in-depth review articles on specific topics. While LD is a global public health concern across the Northern Hemisphere, this review article is largely USA-centric in terms of manuscripts discussed since aspects of LD vary geographically, including the primary causative agents and their vectors; pathogenicity and common disease manifestations; and the prevalence and incidence of disease.
Clinical and Translational Medicine
Next, we review progress in the diagnosis and treatment of LD, including emerging diagnostic assays and novel therapies. We also describe 2-well-defined subgroups of patients with post-treatment sequelae, including those with antibiotic-refractory Lyme arthritis (LA) and PTLD.
The diagnosis of LD can be a complex task for the provider because, outside of the erythema migrans (EM) lesion of early LD, diagnosis relies on non-specific clinical signs and symptoms that may or may not be supported by laboratory evidence. A prospective study evaluated the ability of emergency room (ER) physicians across 5 hospitals in endemic areas in the Northeast of the USA to accurately discriminate between LD (early disseminated or late) and non-LD using clinical judgment alone, prior to the receipt of laboratory evidence. Among 1,021 children being evaluated for LD (based on presence of one or more EM lesions or Lyme serology tests ordered and compatible symptoms) and enrolled in the study between 2015 and 2017, clinician suspicion of LD in the ER setting was found to be minimally accurate compared to diagnoses supported by laboratory evidence. Twelve percent of patients whom the treating clinician deemed to be unlikely to have LD, actually had LD. Thirty-one percent of patients whom the clinician deemed very likely to have LD, actually did not have LD ( 8 ). A true case of early disseminated or late LD was defined in this study as those with compatible symptoms and positive two-tier serology per guidelines, the limitations of which are reviewed below. The accuracy of clinician assessment of patients presenting with a single EM lesion ( n = 42) was not assessed. The challenges of discriminating between an EM lesion of LD and a non-EM lesion are included below, along with a description of a novel imaging tool that may aid clinician assessment of this sign of LD ( 9 ).
Many patients struggle with getting a timely diagnosis and treatment for LD. Around 40% of patients diagnosed with LD have signs and symptoms associated with disseminated or late LD, indicating that delayed diagnosis and treatment are a common occurrence ( 10 ). In a recent population-based study of 778 patients surveyed in Pennsylvania who were treated for LD in the past 5 years, 31% had a time to treatment >30 days and 10% had time to treatment >6 months, where time to treatment is defined as the sum of time to first medical contact and time under care until receiving treatment ( 11 ). A qualitative study of 26 patients treated for LD in Pennsylvania suggests that patient appraisal of their own signs and symptoms plays a role in delayed treatment, specifically the misattribution of non-specific symptoms, the intermittent nature of symptoms and the lack of a “bull's-eye rash,” which is commonly misunderstood to be the only representative skin lesion of LD ( 12 ). High rates of initial delayed or misdiagnosis is also commonly reported by LD patients that meet the PTLDS case definition ( 13 ) or those with chronic symptoms more broadly ( 14 ).
The consequences of the diagnostic challenges in LD are potentially significant for patients and may lead to missed or delayed diagnosis and exposure to inappropriate or inadequate treatment. Next, we review some recent findings and the current challenges related to the diagnosis of LD.
Exposure to Ticks
The collection of tick exposure history from patients suspected of having LD lacks sensitivity because ticks are stealth biters. They are able to avoid detection by human hosts during feeding. Many people diagnosed with LD have no recollection of being bitten by a tick ( 15 ). While the major endemic regions in the USA are the Northeast ( 16 ), mid-Atlantic and upper Midwest states, Ixodes spp. ticks capable of carrying LD pathogens are found in many states. A recent citizen-science based effort to collect ticks submitted by volunteers from across the USA identified ticks capable of carrying Borreliella species in 35 states ( 17 ). In California, where LD is not considered endemic, infected Ixodes spp . ticks have been found in 42 counties (72%) according to surveillance data ( 18 ).
Serological Testing
Two-tiered serological testing is widely used to support the diagnosis of LD. The two-tiered testing algorithm consists of a first-tier enzyme immunoassay (EIA) or ELISA, and for samples that are positive or equivocal (borderline) on the first tier, a second tier immunoblot is performed. Using the CDC's algorithm, the immunoblot is positive if at least 2 of 3 bands are present on the IgM immunoblot within 30 days of symptom onset or 5–10 bands are present on the IgG immunoblot at any time ( 19 ). A modified two-tiered testing algorithm was approved by the CDC in 2019, which uses a first-tier ELISA, and instead of the confirmatory immunoblot, uses 1 or 2 additional ELISAs that target different antigens than the first-tier ELISA ( 20 ).
Serology presents several challenges for accurate diagnosis. The human body takes time to generate anti- Bb antibodies, so serological testing is not sensitive during early infection ( 21 – 24 )—the period when treatment is most likely to succeed. For patients with disseminated LD or later manifestations such as late Lyme arthritis, serological testing has improved performance compared to early disease ( 22 , 25 , 26 ). However, current tests also lack sensitivity following antibiotic treatment of acute LD, as seroconversion occurs less frequently ( 21 , 23 , 27 – 30 ). Rebman et al. ran two-tier serology on acute and convalescent sera samples collected from 104 patients with clinician-diagnosed EM rash and 21 days of antibiotic treatment. They observed 41 (39.4%) of these patients were seronegative at both the acute and convalescent time points; only 7 (6.7%) patients were observed to have IgG seroconversion at either time point ( 29 ). Seroconversion was also rare in samples from the Lyme Disease Biobank, where only 3 of 83 samples (3.6%) from patients with EM > 5 cm seroconverted at the convalescent draw (2–3 months after the acute draw) ( 23 ). Other widely known limitations of serological tests are that they are unable to distinguish between a prior exposure to Bb and an active infection, may cross-react with non- Bb antibodies, are subject to variable results depending the selection of antigens used in the first-tier test ( 31 ) and some assays, especially the Western immunoblot, require interpretation that may introduce bias ( 32 , 33 ).
For patients with an EM > 5 cm in an endemic area with a history of tick exposure, a clinical diagnosis is sufficient ( 34 ). Testing is not indicated in these patients, and the serologic tests would likely be negative due to lack of antibody development in early disease. For patients with early LD presenting without EM, diagnosis is incredibly challenging.
Signs and Symptoms
If untreated, a patient with a Bb infection may go through several stages of LD, with different signs and symptoms at each stage [reviewed in ( 35 )]. In most people, the first stage of LD begins with “flu-like” symptoms and an EM lesion. LD is known as the “great imitator” because symptoms are varied and often overlap with common health complaints, sometimes making early diagnosis more difficult ( 36 , 37 ). The most common symptoms of early LD are fever, chills, headache, fatigue, neck stiffness, myalgia, joint pain and swollen lymph nodes. There are likely hundreds of health conditions with significant overlap with these non-specific signs and symptoms. As spirochetes disseminate from the site of the tick bite, additional EMs and manifestations can occur including 7th cranial nerve palsy, meningitis, or Lyme carditis [reviewed in ( 38 )]. In the third stage, without proper treatment, patients may also experience neuroborreliosis or Lyme arthritis (LA).
The type and severity of LD manifestations are known to vary across infected individuals for reasons that are unclear but are likely attributable to both, differences in the infecting pathogen and the characteristics of the infected individual. They range from asymptomatic or subclinical infection ( 39 – 41 ) all the way to severe complications from LD that, in rare cases, result in death from Lyme carditis ( 42 , 43 ).
The characteristic EM lesion develops inconsistently across humans 3–30 days following a bite from an infected tick ( 44 ). The EM is often an annular, erythematous, expanding cutaneous lesion that may or may not have a central clearing. While it is sometimes referred to as a “bulls-eye rash,” presentation is known to vary considerably ( 15 , 45 – 47 ). Variation in skin pigmentation, as well as coloring and shape of the rash may also lead to missed or delayed clinical diagnoses ( 48 ). The central clearing in the rash is reported to be less common in endemic areas compared to non-endemic areas ( 15 ). While reports vary across studies, up to 30% of individuals diagnosed with LD do not develop an EM lesion ( 47 , 49 – 52 ) or its presence is missed. If an EM lesion is absent, there is no clinically recommended laboratory test available to aid in the diagnosis of early LD because the currently recommended serologic tests are highly insensitive in the first few weeks of infection ( 51 ).
Direct Detection of Bb
Many bacterial infections are diagnosed using a variety of culture methods and the confirmation of pathogen identity through molecular techniques or differential biochemical assays ( 53 , 54 ). This is not currently practical or feasible for LD. The direct detection of the pathogen in blood can be a challenge because of the narrow window of spirochetemia that is more likely during early infection and the low numbers of circulating Bb ( 55 , 56 ). While the pathogen may disseminate from the site of the tick bite through the blood, it also disseminates through the lymphatics and is known to invade other more privileged tissues, such as the heart, nervous system, and connective tissue. Bb is a fastidious, slow-growing bacteria that requires up to 12 weeks of incubation in culture before a negative result is determined, which is too long to be useful in clinical diagnosis ( 32 ). In one study on the ability to detect spirochetemia in patients with EM through culture methods, they estimated 1 cultivable spirochete per 10 mL of whole blood ( 55 ). Bb culture also requires specialized skills and tools that most laboratories are not equipped with outside of the research setting, where these techniques remain valuable for basic science research ( 21 ). Finally, antibiotic treatment decreases culture positivity rates, making it useful only in untreated patients ( 21 ). Blood, serum or plasma is not a reliable tissue to detect Bb by PCR because the spirochetes are transient and in low copy number. Skin biopsy from the EM lesion is a more useful tissue diagnostically, but this step is invasive and patients that present with an EM lesion do not require laboratory confirmation for diagnosis of LD ( 21 ).
Emerging Diagnostics
For the reasons outlined above, there is an urgent need for pathogen-detection methods that are highly sensitive and specific and capable of reliably detecting infection by multiple pathogenic species of Borreliella and strains of Bb (see section Genomic Insights From Borreliaceae Lineages) at all stages of infection and disease ( 57 ). Of special concern are individuals with acute infection that do not present with an EM rash and have yet to generate a humoral response to Bb . The factors that control the development of EM rash also need to be delineated, along with a surrogate set of biomarkers to aid diagnosis of more complex cases of suspected LD. Ixodes ticks can carry multiple human pathogens (see section Transmission of Bb via Ixodes spp. Vectors), and diagnostic methods capable of detecting the most prevalent tick-borne pathogens and clinically relevant strains of Borreliella are needed. Promising new diagnostic methods are being developed using serology, direct detection assays, and other tests that measure host response to the pathogen. Select assays are outlined in Table 2 . There is also a significant unmet need for diagnosing PTLD. Currently, this diagnosis is performed, in part, based on self-reporting of symptoms. Several groups are investigating correlation between blood transcriptome; blood metabolome; and gut microbiome of PTLD patients in search of potential diagnostic and causal markers. Of note are recent studies describing a distinct microbiome signature ( 58 ) and a blood metabolome signature ( 59 ).
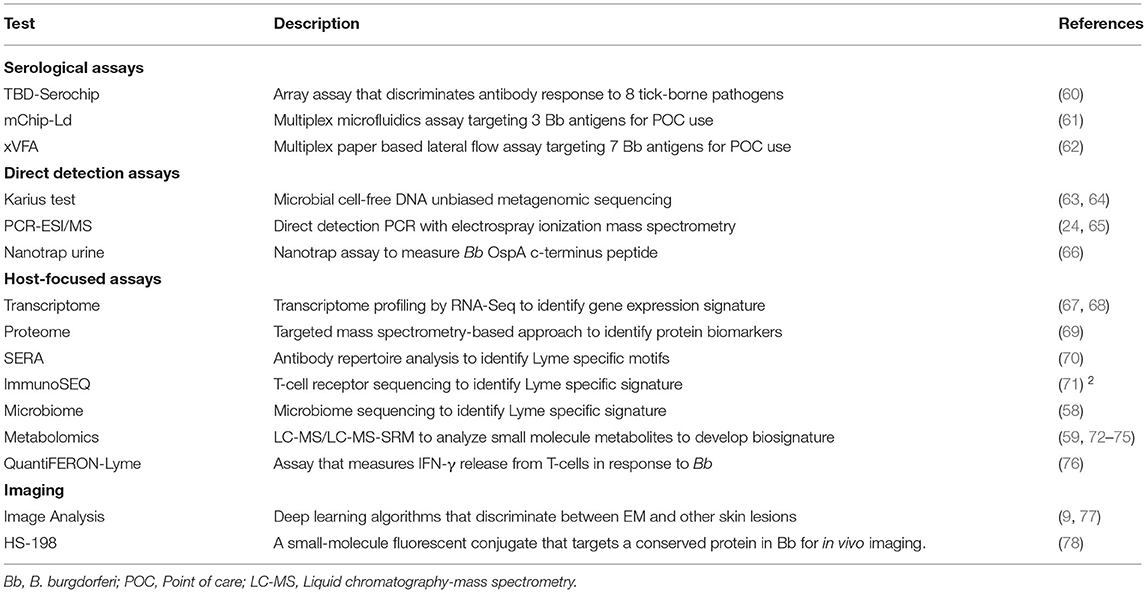
Table 2 . Emerging diagnostic assays for LD.
Recommended Treatment
Antimicrobial therapy for LD is often successful, especially when patients are treated in the early phase following detection of an EM lesion ( 79 ). As disease progresses, treatment must be extended and may be less effective ( 80 , 81 ). Administration of doxycycline or amoxicillin for 14–21 days is the recommended treatment for early or early disseminated phase patients who do not have neurological involvement ( 44 ). Lyme arthritis is an indication of disseminated disease and the recommended treatment for this is the aforementioned oral antibiotic for 28 days. For patients with clinically evident neurological involvement, treatment with intravenous ceftriaxone is recommended. These suggested regimens are based on objective measurements. However, in the guidelines from the Infectious Disease Society of America (IDSA), the authors point out that “Response to treatment is usually slow and may be incomplete” ( 82 ).
There is widespread agreement in the medical community about the appropriate treatment of acute LD ( 83 ), however the appropriate treatment of patients meeting the Post-treatment Lyme disease syndrome (PTLDS) case definition remains a challenge due to incomplete knowledge about the condition and related uncertainties. Moreover, the recently updated IDSA guidelines for the treatment of LD remove mention of PTLDS altogether ( 83 ).
More clinical trials are needed to assess the efficacy and safety of drug regimens and complementary therapies for LD and its sequelae. This year, the first clinical trial coordinating center was established at Columbia University to facilitate the conduct of high-quality multi-site clinical trials and pilot studies related to LD and other TBDs. 1 Furthermore, tools capable of discriminating the etiology of persistent health issues following treatment for LD are needed in order to improve therapy development efforts and target treatments for more precise patient-centered care.
Drug Discovery and Preclinical Studies
Although early-stage LD can be successfully treated with doxycycline or amoxicillin, late-stage LD with arthritis and neurological symptoms can be refractory to antibiotic treatment. Wu et al. showed that stationary phase Bb are unexpectedly susceptible to cell wall synthesis inhibitors, and vancomycin in particular almost completely eradicates persisters in vitro ( 84 ) (see section Persistent Infection). Feng et al. recently identified FDA-approved drug candidates that are more effective at killing dormant Bb persisters in vitro than the current Lyme antibiotics ( 85 ).They found the drug combination Daptomycin + Cefoperazone (or Cefuroxime) + Doxycycline was most effective at eradicating Bb grown in vitro and more recently in the mouse model for Bb persistence ( 86 , 87 ).
Complementary Therapies
Zhang et al. recently identified natural products and botanical medicines ( Cryptolepis sanguinolenta (Yellow dye root) , Juglans nigra (Black walnut), Polygonum cuspidatum (Japanese knotweed), Uncaria tomentosa (Cat's claw), Artemisia annua (Sweet wormwood), Cistus incanus , and Scutellaria baicalensis (Chinese skullcap) with good activity against both stationary phase and growing Bb ( 88 ). More recently, botanical medicines Cryptolepis sanguinolenta, Artemisia annua, Scutellaria baicalensis, Polygonum cuspidatum , and Alchornea cordifolia have also been shown to have activity against Babesia duncani ( 89 ). Since the above herbal medicines that have activity against Bb and Babesia have been used traditionally in patients with good safety profile, proper clinical trials are needed to evaluate their utility in treating patients with LD and coinfections. In addition, some essential oils such as oregano, cinnamon bark, clove bud, citronella, garlic, allspice, myrrh, hydacheim, and Litsea cubeba were shown to have excellent activity against both stationary phase and growing Bb ( 88 , 90 ). However, further studies are needed to evaluate the active compounds in the essential oils, in order to elucidate the specific activity, to assess their potential toxicity and pharmacokinetic properties for activity against Bb infection in animal models before human use.
Well-Defined LD Patient Subgroups With Posttreatment Sequelae
Antimicrobial therapy effectively treats LD in most people, however some patients experience ongoing health issues following treatment ( 4 , 91 ). Antibiotic-refractory LA is the most well-characterized subgroup of patients with posttreatment sequelae. In recent years, characterization of patients with PTLD, a well-defined subset of patients with persistent or chronic LD, has also advanced considerably. Next, we review progress in the description of each of these post-treatment sequelae.
Antibiotic Refractory LA
The LD patient subgroup with LA has been meticulously studied for decades. In 1975, a cluster of cases was brought to the attention of the Connecticut State Department of Health independently by two mothers concerned about the number of children with swollen knees with similarities to juvenile arthritis, a rare condition. An investigation revealed 51 similar cases (39 of these children) in Old Lyme, Connecticut and adjacent townships ( 92 ). By 1983, the causative agent and vector of LD were identified ( 93 ). LA—the proliferative and persistent synovitis of one or more large joints—is the most prevalent symptom in late-stage LD patients [reviewed in ( 94 )]. LA may wax and wane months to years following untreated infection ( 95 ). Around 10% of LA patients experience persistent LA that does not resolve within a couple months after one or more rounds of antibiotic therapy ( 95 – 97 ). LA patients refractory to oral antibiotics are treated with intravenous ceftriaxone antibiotic therapy, with mixed results in adults ( 97 , 98 ) and children ( 99 ). Persistent LA despite aggressive treatment with antibiotics is referred to as antibiotic refractory LA ( 100 ), or if no evidence of ongoing infection, post-infectious LA ( 101 ). Among patients with persistent Lyme arthritis, determining whether persistent joint inflammation is due to an ongoing antibiotic-refractory infection or a post-infectious immune response also raises important treatment dilemmas that are not yet resolved, as reviewed elsewhere ( 95 ). The true cause of antibiotic-refractory LA has remained enigmatic. Recent developments, however, suggest a complex interplay between both bacterial and host factors ( 102 ).
What bacterial components may contribute to inflammation after therapy? While the debate surrounding persistent infection continues (see section Persistence), undetectable levels of bacteria in the SF of some patients with antibiotic refractory LA has led to a search for alternative explanations for persistent inflammation. Intravital microscopy studies of antibiotic treated mice observed the persistence of Bb -derived material ( 103 ), which precipitated the possibility that bacterial debris may be responsible for immune activation. Later, we highlight a recent finding that a component of the bacterial cell envelope may exacerbate and prolong the initial response to the LD agent (see section Persistent Antigenic Debris).
PTLD is a narrow but defined subset of patients with persistent symptoms following treatment for LD [reviewed in ( 30 , 44 )]. Patients with PTLD suffer persistent or relapsing symptoms, such as severe fatigue, cognitive issues, sleep disturbance, and musculoskeletal pain that negatively impact their functional abilities at least 6 months following treatment for LD. The reductions in quality of life for patients with PTLD are comparable to patients suffering from congestive heart failure ( 104 , 105 ). These health problems can last months or years following the initial treatment for LD. The incidence of PTLD is as high as 20% in some patient populations ( 13 , 44 , 91 , 106 – 108 ). Prevalence of PTLD is currently difficult to ascertain, due in part to the variability in the case definition applied in the literature and variability in reported rates of treatment failures among patients with LD, so estimates range from 69,000 to more than 1 million cases in the USA ( 107 , 109 ).
Descriptions of patients with persistent symptoms following antibiotic treatment for LD date back to studies in the 1980's ( 110 , 111 ) and 1990's ( 79 , 80 , 112 , 113 ). The term “post-treatment [chronic] Lyme disease syndrome” and a case definition PTLDS were first described in 2003 ( 114 ) and 2006 ( 44 ), respectively. Today this case definition, along with standardized measurement of subjective symptoms ( 115 ), informs the eligibility and exclusion criteria for many studies and trials related to the broader and more heterogeneous population with chronic or persistent Lyme ( 91 , 116 , 117 ), so that outcomes from research studies may be compared and potential mechanisms of pathogenesis defined with less ambiguity ( 44 ). Recent studies have deployed deep phenotyping methods and identified multiple biomarkers that distinguish these patients from LD patients that return to health and/or controls, as described below. These studies provide evidence that PTLD is an infection-associated condition with a distinct biology. Here and throughout, we adopt the terminology PTLD, as previously proposed ( 44 , 118 ), to describe this condition.
The diagnosis and treatment of patients with PTLD face significant challenges. Objective tests to diagnose PTLD currently do not exist. Instead, diagnosis is made clinically through a process of documenting a prior history of LD and excluding other potential causes of persistent symptoms. Currently there are no prognostic indicators to stratify LD patients for their susceptibility to PTLD, but elevated levels of the T-cell chemokine CCL19, IL-23 or muted B-cell response were recently reported to be more common among PTLD patients compared to those that return to health following initial treatment ( 119 – 121 ). For patients that progress to PTLD, there are no FDA approved curative therapies. Safety and efficacy data for off-label treatment regimens and complementary therapies for PTLD are not well-established. Objective tests to determine whether a patient is cured or responsive to therapy also do not exist.
Objective laboratory tests that improve the diagnosis and treatment of this patient subgroup appear to be on the horizon in part due to investments in the creation of carefully constructed cohorts and biorepositories of well-defined patients with PTLD (see section Biorepositories and Research Cohorts). For example, the first biomarker of PTLD to be identified in one cohort that was then confirmed in a second, independent cohort of PTLD patients in the USA was published in 2020. This metabolomic signature discriminates clinically cured LD patients without PTLD from LD patients with PTLD ( 59 ). Alteration of gene expression was shown to persist after treatment for early LD among a small sample of patients followed longitudinally for 6 months. While no transcriptome signature was identified that could discriminate between patients that return to health after treatment compared to those that do not, the signature may lead to new objective tests for early LD ( 67 ). A gut microbiome signature was also recently identified that could distinguish PTLD patients from both healthy controls and ICU patients, further suggesting that PTLD is a distinct and definable disorder even if the underlying etiology remains uncertain ( 58 ). Other biomarkers related to LD and PTLD are reviewed below (section Pathogenesis). Taken together, these findings indicate that novel diagnostics and treatments for PTLD are now within reach.
Fundamental Knowledge
Genomic insights from borreliaceae lineages.
Between 1982 and 2010, the B. burgdorferi species complex, known as B. burgdorferi sensu lato , steadily expanded from 1 to 18 species (sometimes referred to as genospecies) as isolates from tick vectors, their hosts, and patient samples were characterized [reviewed in ( 122 )]. A subset of these species are associated with human disease. B. burgdorferi sensu stricto (Bb) in the USA, as well as B. afzelii and B. garinii in Eurasia are the most common agents of LD in the Northern hemisphere. Cases of LD in Europe are also caused by Bb and B. bavariensis ( 123 ), but are less common. B. spielmanii ( 124 ), B. bisettiae ( 125 – 127 ), and B. lusitaniae ( 128 , 129 ) have been identified in human specimens but their clinical importance is less clear. B. valaisiana has been identified in human specimens ( 130 ), but others have recently provided compelling reasons why existing evidence does not support it being considered a human pathogen ( 131 ). Additional species have been identified in tick vectors or their hosts, but not in patient samples.
The genus Borrelia was recently divided into two genera ( 132 , 133 ). This division groups the tick- and louse-borne relapsing fever (RF) agents and their relatives into the genus Borrelia and the LD agents and their relatives into the genus Borreliella . Historically, Borrelia species were classified according to whether they were transmitted by hard-bodied or soft-bodied ticks ( 134 ). The advent of molecular characterization through genetic analyses, and more recently, whole genome sequencing, has led to a refinement in our ability to classify spirochetes that are morphologically very similar. The division of the genus is based on phylogenetic and comparative genomic analyses using 38 public genomes of 18 different species. Due to naming standards, RF agents retain the genus Borrelia since they were the first species identified in the genus. The reclassification of Borrelia burgdorferi sensu lato species to the genus Borreliella means that their names will change but the abbreviations are retained, e.g., B. burgdorferi for Borreliella burgdorferi (Bb) . The proposal generated considerable debate across the scientific community ( 135 , 136 ). While adoption of the new naming convention has thus far been low in recently published literature, canonical bacterial reference manuals ( 133 ) and scientific databases have begun to incorporate the change.
New clinically relevant Borreliella species continue to be discovered [reviewed in ( 134 , 137 ), reviewed in ( 138 ), reviewed in ( 139 , 140 )]. In 2016, a new pathogenic species, B. mayonii , was identified in the upper Midwest of the USA through PCR analysis of more than 100,000 human tissue specimens collected between 2003 and 2014. Specimens from a total of six patients were deemed positive for B. mayonii , all presenting after 2012 with signs and symptoms consistent with LD ( 141 ). The genomes of two B. mayonii isolates have been sequenced, with notable differences from Bb (strain B31) including the absence of the complement inhibitor Cszp and dozens of other proteins ( 142 ).
Bb has one of the most complex genomes of any bacteria characterized to-date [reviewed in ( 143 )]. Bb contains a single linear chromosome of ~900 k base pairs (bp) with between 7 and 21 different plasmids, ranging in size from 5 to 84 kbp as reviewed from the genome sequences for 27 Bb isolates determined since the elucidation of the Bb B31 genome sequence in 1997 ( 144 ). The single chromosome appears to be very constant in gene content and organization across these Bb isolates. Overall, dozens of Borreliella isolates have been sequenced ( 144 – 147 ). These include the partial genomes of 64 isolates recently identified from collections across Canada, an emerging area for LD ( 148 ). To-date, chromosome assemblies have been reported from these isolates without, however, an equivalent analysis of the plasmid content. In the Bb genome, the conserved linear chromosome encodes most housekeeping genes, while the variable set of linear and circular plasmids encodes most of the outer membrane lipoproteins ( 149 – 151 ). Three plasmids, cp26, lp17, and lp54, are conserved across sequenced isolates of Bb to-date [reviewed in ( 143 )]. The content and number of the plasmids however appear much more variable with some loss of these once Bb is in vitro cultured for an extended period of time. It is noteworthy that while extra-chromosomal DNAs are often referred to as plasmids—non-essential DNA that carries pathogenic and/or material that infer a selective advantage in a particular situation—some Bb “plasmids” carry essential genes and are more akin to mini-chromosomes. Knowledge about plasmids is currently limited due in part to the constraints next-generation sequencing technologies impose with short reads and the subsequent challenges with assembly. Long-read sequencing technology is poised to enhance our understanding of plasmid content and their dynamics across species and strains ( 150 , 152 ).
Characterization of Borreliella isolates at the genome level is important to determine how genomic variation correlates with different disease phenotypes [reviewed in ( 122 , 157 )]. Molecular typing is used to classify distinct lineages of Borreliella . Common methods include pan-genome snp analysis ( 153 ), multi-locus sequence typing (MLST) ( 154 ), ribosomal RNA intergenic spacer typing (RST), and outer surface protein C (OspC) typing [( 150 ), reviewed in ( 155 )]. These methods for the classification of strains of Bb yield different numbers of distinct groups. Isolates of Bb from clinical samples collected from patients in the USA and Slovenia were recently compared and distinct differences were observed across genotypes, clinical manifestations, and inflammatory potential ( 156 ). In another recent study, serial blood samples obtained over a 21 day period from four patients with acute LD were assessed for infection with Bb via a novel direct detection method that combines PCR and electrospray ionization that is also able to discriminate different Bb genotypes. Two of 4 patients were infected with more than one genotype of Bb . Notably, the dominant Bb genotype changed over time in these two patients during antibiotic treatment ( 65 ). Infection with heterologous strains of Bb in humans is consistent with studies of pathogen burden in both ticks and vertebrate hosts (see section Transmission of Bb via Ixodes spp. Vectors). New technologies and high-throughput screening methods are advancing our understanding of which genes in the Bb genome are critical for ecologically or clinically relevant phenotypes, such as infectivity, tissue tropism ( 157 ) or drug tolerance. In a series of recent experiments, transposon insertion sequencing (Tn-seq) was used to identify genes associated with mammalian infection, resistance to oxidative stress, and survival in tick vectors ( 158 – 160 ).
LD agents are believed to inflict damage to people through inflammation caused by their immune response. No exotoxins have been identified in the genome with similarity to any previously described exotoxin found in other bacterial species, and the necessary secretory components appear to be absent. In addition, unlike most classical diderms, Bb does not produce the endotoxin Lipopolysaccharides (LPS) ( 144 ). A genome-wide proteome screen for immunogens in Bb (B31) using sera from patients with natural infections found that about 15% of the 1,292 open reading frames evaluated code for products that are immunogenic ( 161 ). More than 120 lipoproteins are encoded in the Bb genome ( 162 ) and represent nearly 8% of open reading frames ( 163 ). Of 125 lipoproteins examined experimentally, 86 of these are secreted to the bacterial surface ( 162 ).
Proteomic Insights From Borreliaceae Lineages
The application of mass spectrometry-based proteomics has recently gained interest in the field of Bb and LD research to advance the understanding of disease pathogenesis and develop potential diagnostic methods and vaccines. Progress in Bb proteomics has been limited primarily to the proteins contained in the chromosome, while proteins encoded in the extra-chromosomal plasmids remain poorly characterized. In either case, attention has also been focused substantially on proteins encoded by genomic sequences of Bb that are homologs of B31—the vastly used isolate that was first collected from Shelter Island, New York ( 164 ). Further, very few laboratories working on proteomic workflows generate high-quality genomic data from different isolates of Bb . Nevertheless, advanced mass spectrometry-based proteomics provide unmatched information such as protein identification, quantification and post-translational modifications. The characterization of the Bb proteome enables basic science research and the development of vaccines and diagnostics ( 44 , 165 ). Though several proteomic studies have been published, no high-resolution mass spectrometry (MS) data is represented in a publicly accessible repository for the community to access and utilize in research goals ( 162 , 166 , 167 ). The recent construction of the Borreliella PeptideAtlas repository ( http://www.peptideatlas.org/ ) provides a unique community resource that contains large-scale assembly of observed and MS-derived validated data uniformly processed through the Trans-Proteomic Pipeline (TPP) ( 168 ). This database contains the proteome information from Bb isolates B31, 5A4, 297 and MM1 where 39,145 distinct peptides are validated and represent 1,283 Bb proteins. In addition to the unique peptide identification data, post-translational modifications are also presented with their validated MS spectra. The Borreliella PeptideAtlas is a dynamic proteome resource in terms of size and complexity and is continually updated as new datasets of Bb proteomes become available. These new datasets are ingested, processed through the TPP data analysis pipeline to ensure low false-discovery rates and presented in the conglomerated relational database for all users to explore and utilize. For example, the data can be mined to provide leading candidates across the isolates represented and the experimental conditions they were subjected to allowing exploration of how Bb adapts to, and is able to survive in a wide variety of environmental conditions. This resource provides a foundation for researchers to understand the dynamics of proteome organization throughout stages of infectivity and to generate targets to arrest infectivity.
Transmission of Bb via Ixodes Spp. Vectors
The black-legged ticks, Ixodes scapularis and Ixodes pacificus on the West Coast, are the primary vectors of Bb in the USA. In endemic areas, the proportion of Ixodes spp . ticks infected with Bb can be remarkably high. One recent survey of the pathogen burden of 197 Ixodes scapularis ticks collected from New York and Connecticut where LD is endemic, revealed 111 (56%) ticks were infected with Bb and 37 (19%) were co-infected with more than one human pathogen ( 169 ). The high pathogen burden is consistent with previous tick surveys in the same region ( 170 ). In contrast, the percent of infected ticks in other regions of the USA is much lower. In recently published surveys across California, for example, fewer than 5% of Ixodes spp. ticks were infected ( 17 , 170 ). Ixodes ticks sometimes carry multiple strains of Bb ( 65 , 150 , 171 – 173 ) that may impact the course of disease in people that are co-infected. One tick survey showed that 39% of Ixodes ticks in North America are infected with multiple genotypes of Bb ( 170 ).
Ixodes scapularis ticks feed only once per life stage (larvae, nymph, adult) after hatching from eggs. They primarily acquire Bb through feeding on infected vertebrates that are mostly mammals, but also some species of birds ( 174 ). Bb spirochetes colonize and persist in the tick until some are transmitted to a new host during the next feeding, while others remain in the tick during molting and the next life stage ( 175 ). Bb spirochetes are not transmitted from the adult tick to the egg, or it may occur only rarely. While transovarial transmission (TOT) of Bb in Ixodes spp . ticks has been reported in the literature, these reports may be attributable to confusion between B. miyamotoi , a relapsing fever agent, and Bb ( 176 , 177 ).
Bb spirochetes have evolved multiple mechanisms to aid survival in adverse environments throughout the enzootic cycle. While colonizing the tick gut they must impede immune defenses of the tick, those of the host blood meal, and coexist with other residents of the tick gut microbiome. After their migration from the tick gut through the tick body cavity (hemocoel) and into the tick salivary glands, Bb spirochetes are expelled during feeding into the next host where they must again impede immune defenses and change their gene expression to survive and establish infection in a vertebrate host. The diverse and dynamic interactions between Bb and Ixodes ticks during Bb colonization, persistence, and transmission was recently reviewed elsewhere ( 178 ), but several recent findings are worth highlighting here.
The stability, abundance and diversity of the tick gut microbiome in Ixodes spp. is unsettled ( 179 , 180 ), but there is evidence that the microbiome impacts the ability of Bb to effectively colonize the tick gut ( 181 ). The Bb genome lacks interbacterial defense pathways, which suggests they may not thrive in polymicrobial environments and the presence of certain taxa may interfere with colonization and persistence in the tick gut. For example, Pseudomonas possess genes for a Type VI secretion system that can deliver toxins to bacterial and eukaryotic members also residing in the tick gut microbiome ( 179 , 182 ). Consistent with these findings, ticks infected with Pseudomonas are associated with lower burden of Bb ( 179 , 182 ). Future research on the tick microbiome may lead to insights about approaches to disrupt pathogen colonization and transmission.
The tick salivary glands are important for transmission of Bb spirochetes. Tick saliva is essential to adequate tick feeding and contributes to human infection [reviewed in ( 183 ), reviewed in ( 184 ), reviewed in ( 185 )]. During feeding, a tick alternates between secreting saliva and ingesting blood meal. Tick saliva not only carries pathogens from the tick into the host, but also contains molecules that function to aid the feeding process by creating blood flow through vasodilation and anticoagulative properties ( 186 ), blocking pain and itch ( 187 , 188 ), impeding wound healing ( 189 ), and suppressing host immune response ( 190 , 191 ). The composition of proteins expressed in tick saliva changes during feeding, which suggests another potential mechanism for host immune evasion through antigenic variation ( 192 ). A greater understanding of the components of tick saliva that support the infectivity of Bb may provide new routes to the prevention of LD.
Pathogenesis
The pathogenesis of LD is believed to be driven in large part by the immune response of patients, although the underlying causes of ongoing inflammation and tissue damage in different stages of LD remain an active area of research. The microbial origin and inflammatory nature of untreated LD is better understood than the pathophysiology that underlies persistent signs and symptoms of disease experienced by some individuals following antimicrobial treatment. The dominant hypotheses about potential mechanisms underlying PTLD are immune inflammation and dysregulation and persistent infection and/or persistent antigenic debris. In this section, we review progress in the past 5 years on our understanding of pathophysiology of LD, starting with untreated LD. Then, we will review new evidence that has emerged related to persistent disease following treatment.
Immune Activation and Inflammation in Untreated LD
Inflammation is an important mechanism the body uses to aid in the elimination of pathogens. However, too much inflammation, such as what happens during a “cytokine storm” among some severe COVID-19 patients when cytokines are overproduced, can overwhelm the body and cause grave tissue injury. An aberrant inflammatory response is also what underlies autoimmune disease ( 193 ). In this section we review immune activation and inflammation associated with LD that begins soon after an infection with Bb from a tick bite.
The skin is an important first barrier to Bb infection. Interactions between the pathogen and human skin begin at the site of the tick bite where Bb spirochetes invade then disseminate outwardly, sometimes causing circular or elliptical EM lesions in their wake. One study induced suction blisters over EM lesions of LD patients in order to characterize the dermal leukocytes and cytokines of the aspirates from the skin. They found the aspirates to be enriched for T cells, monocytes/macrophages, and dendritic cells (DCs) compared to uninfected controls. Two cytokines, IL-6 and INF-γ, were predominant in the EM lesions ( 194 ).
The response of the innate immune system, including complement pathways and acute-phase proteins (APPs), occurs more or less immediately while the adaptive B- and T-cell response may take days to weeks. Macrophages and dendritic cells resident in the skin express a range of pattern recognition receptors (PRRs) that initiate signaling cascades and inflammatory responses as an early line of defense when they encounter and internalize a Bb spirochete [reviewed in ( 195 )]. A yeast display screen of >1,000 extracellular and secreted human proteins identified direct interactions between Bb isolates and one human host factor, Peptidoglycan Recognition Protein 1 (PGLYRP1). In vitro assays show recombinant PGLYRP1 binds with purified peptidoglycan from Bb and have borrelicidal activity ( 196 ). In murine models deficient in PGLYRP1 (PGLYRP1 −/− ), pathogen burden of experimentally infected mice were higher than wild-type in hearts and joints but not skin. Moreover, PGLYRP1 −/− mice had reduced IgG serum levels and elevated proinflammatory cytokines (IFN-γ, CXCL9, CXCL10). The relationship between PGLYRP1 and pathophysiology of LD in humans is not yet known.
Phagocytic cells will attach to a spirochete via a repertoire of host cell surface receptors. These include Complement Receptor (CR) 3 and CD14 ( 197 – 200 ), urokinase receptors (uPAR) ( 200 , 201 ), scavenger receptors such as macrophage receptor with collagenous structure (MARCO) ( 200 , 202 ), some Toll-like receptors (TLRs), C-lectins, sialic acid-binding immunoglobulin-like receptors (siglecs), Fc receptors, or others ( 200 ). The attachment of Bb to host cell surface receptors leads to signaling that induces innate and specific adaptive immune responses, as well as clearance of the pathogen through phagocytosis. For example, the host cell surface receptor, Toll-like receptor 2 (TLR2), is a PRR that binds to ligands on the Bb cell surface that display certain pathogen associated molecular patterns (PAMPs). When a PRR senses a pathogenic ligand, it upregulates an inflammatory response, which commonly includes the induction of pro-inflammatory cytokines, chemokines, and antimicrobial peptides (AMPs); however, different types of activated PRRs induce different gene expression patterns. The production of pro-inflammatory cytokines, such as interleukin-6 (IL-6), recruits blood cells to the site of inflammation and induces the production of APPs, such as C-reactive protein (CRP).
The internalization and degradation of engulfed Bb spirochetes is sensed by intracellular receptors, including the NOD-like receptors (NLRs) and endosomal TLRs. These receptors activate signaling pathways that induce the production of cytokines, including interferons (IFNs). These are classified into three main types: I (IFN-α or IFN-β), II (IFN-γ), and III (IFN-λ) [( 203 ), reviewed in ( 204 ), reviewed in ( 205 )]. Type I IFNs are induced by Bb DNA or RNA through TLR7 and TLR9 ( 203 ), as well as TLR8 in monocytes ( 206 ). Type II IFN is produced by innate lymphocytes, including natural killer cells (NK cells) and by T helper cells (Th) ( 207 ). In vitro , type III IFN is induced in peripheral blood mononuclear cells (PBMCs) through TLR7 by live spirochetes or purified Bb RNA ( 208 ), perhaps controlled by Bb plasmid lp36 ( 203 ). The role of the type III IFN pathway in disease pathogenesis has not been fully elucidated, although clinical isolates associated with disseminated disease produce stronger IFN responses (Type I and III) ( 203 ).
The early stages of LD show an elevation of inflammatory markers and immune mediators ( 69 , 119 , 209 , 210 ). CRP is an APP that is used clinically as a marker of inflammation that may denote infection, malignancy or cell stress ( 211 ). Within a few hours of an infection or other stressor, cytokines secreted by immune cells will enter the bloodstream and cause the liver to secrete CRP. Generally, the normal range of CRP for healthy adults is <10 mg/L; moderate elevation is 10–100 mg/L; and marked elevation is >100 mg/L ( 212 ). A longitudinal assessment of CRP levels in serum samples from 44 LD patients presenting with one or more EM lesions and followed for 2 years after treatment, showed a significant elevation of CRP prior to treatment and a rapid decline to control levels following treatment ( 209 ). Elevated levels of CRP at the pre-treatment visit were seen again in a larger study by the same group, along with 6 additional elevated markers, including CCL19, ferritin, fibrinogen, IFN-γ-induced protein 10 (IP-10), monokine induced by IFN-γ (MIG), and serum amyloid A (SAA) ( 119 ). The first systematic study of APP levels in serum samples from patients at different stages of LD and healthy controls was recently conducted ( 210 ). Consistent with the previous studies, CRP levels were most elevated in patients with early localized (single EM, n = 18) and early disseminated (multiple EMs, n = 17) LD, with 33 and 71% of patients, respectively, having CRP levels >10 mg/L ( 210 ). More recently, a proteomic analysis of serum proteins in 70 LD patients with one or more EM lesions at the time of diagnosis, identified six proteins, including CRP, with significantly altered serum levels shared across two independent cohorts ( 69 ). The other five elevated proteins are APOA4, C9, CST6, PGLYRP2, and S100A9. Two independent studies have also shown that the chemokines, CXCL-9 and CXCL-10, both known to be associated with IFN-γ production and thus a “TH-1 type” of immune response, are elevated at time of diagnosis in EM+ LD patients in the USA and Europe ( 120 , 209 ). Among a European cohort, patients with symptoms at study entry had significantly higher levels of CXCL9 compared to patients without symptoms ( 120 ).
Inflammation and Immune Dysregulation Among Patients With Persistent LD
The immune profiles of LD patients with persistent health problems following antibiotic treatment are not consistent across well-characterized subgroups, however several potentially important immune mediators have emerged within subgroups. Next, we will review each of these.
CRP in Patients With Persistent Symptoms Following Treatment
The levels of CRP in other stages of LD (subsequent to early localized or early disseminated, as described above) and patients with persistent signs or symptoms following treatment are less consistent. Among patients with antibiotic-refractory LA ( n = 11), 55% have serum CRP levels >10 mg/L while patients with early neurologic, late neurologic, and antibiotic responsive LA showed no significant difference in CRP levels compared to controls. For patients with PTLD ( n = 74), 15% had CRP levels >10 mg/L compared to 4% of patients with LD that returned to health following treatment ( n = 68) ( 210 ). For some diseases, such as cardiovascular disease, serum CRP in the range of 3–10 mg/L is considered of clinical value for understanding an underlying inflammatory process or stratifying risk in some patients ( 213 – 215 ). A significant proportion of both, antibiotic-refractory Lyme arthritis (73%, n = 11) and PTLD (55%, n = 74) patients have serum CRP levels in this range ( 210 ). While these data are suggestive of an ongoing inflammatory process in these two patient subgroups, more research is needed to better understand the role of CRP, along with other markers of inflammation, and the underlying mechanisms at work in affected patients.
IFN-γ and Antibiotic-Refractory LA Patients
Antibiotic-refractory LA patients without evidence of an ongoing infection, show excessive IFN-γ production. So far, this biomarker appears to be specific for LA as serum levels of IFN-γ are not significantly elevated among EM+ LD patients at the time of presentation compared to controls, nor are levels of this cytokine significantly elevated in patients with PTLD ( 119 ). A recent study did a comparative analysis of synovial tissue from patients with LA, rheumatoid arthritis, and low inflammation osteoarthritis and found that LA is unique, showing elevated levels of IFN-γ and IFNγ-producing T cells and NK cells in synovial fluid ( 216 ). This study also identified and characterized a population of fibroblast-like synoviocytes (FLS) that are hypothesized to be involved in mediating persistent inflammation. These cells, when activated by IFN-γ ex vivo , expressed genes and pathways that overlapped with that seen in postinfectious LA synovial tissue ( 216 ). This suggests that the FLS are driving ongoing inflammation and suppressed wound healing in an IFNγ-dependent manner.
CCL19 Among PTLD Patients
The T-cell chemokine, CCL19, was associated with susceptibility to PTLD. In a recent study, sixty-four cytokines, chemokines, and inflammatory markers were measured in serum collected from 76 EM+ LD patients at six visits over 1 year. Eleven patients (14.5%) went on to develop persistent symptoms that impacted daily functioning following treatment and were classified as having PTLD. Twenty-nine patients (38.2%) had symptoms following treatment without a functional impact on daily living and 36 patients (47.37%) returned to health. Patients with CCL19 above 111.67 pg/ml at the (1-month) visit were 12.6 times more likely to meet criteria for PTLD at the 6 and 12 months timepoints ( 119 ). In a murine model, CCL19 along with IL-23, were associated with a pathological TH17 response in experimental autoimmune encephalomyelitis (EAE) ( 217 ). However, it has not been fully assessed to which extent the TH17 pathway is induced in LD patients.
IL-23 Among European PTLD Patients
A cytokine, IL-23, associated with IL-17 production and thus “TH-17”-type responses, is elevated in early LD and remains so among many European patients that have persistent symptoms following treatment. Eighty-six EM+, untreated LD patients that enrolled in an antimicrobial drug trial in Europe and were followed longitudinally for a year after treatment and were assayed for 26 cytokines and chemokines at 4 time points. Among the patients studied, 45 had symptoms following treatment consistent with having PTLD. One cytokine (IL-23) and two chemokines (CXCL9 and, to a lesser extent, CXCL10) showed significant differences across groups. Most patients with detectable IL-23 levels at study entry went on to develop PTLD and IL-23 levels remained elevated in these patients with ongoing symptoms. The seven patients with the highest levels of IL-23 (≥230 ng/mL) all went on to develop PTLD. Thus, an aberrant TH17-related immune response might be contributing to symptoms in patients with elevated IL-23 ( 120 ). It is interesting to note that no significant difference was identified in serum levels of IL-23 among the longitudinal cohort of LD patients from the USA, while CCL19 was not noted to be significantly elevated in the European cohort ( 119 , 120 ). One potential reason for these differences is the difference in the primary agents of LD in Europe and the USA: B. afzelii and B. garinii vs. Bb . However, the mechanisms underlying these and other differences between LD in North America and Europe remain poorly understood.
Autoantigens and Self-Reactivity in LA Patients
The origin of autoimmune disease (AD) in humans have previously been thought of as triggered by microbial infection, which may serve as a catalyst for development of responses to self-antigens [reviewed in ( 218 )]. Several autoimmune diseases are associated with bacterial triggers, such as gastric autoimmunity and Guillain-Barré syndrome triggered by infection with H. pylori and C. jejuni , respectively [reviewed in ( 219 )]. One proposed mechanism for possible LD-associated autoimmunity is the circumstance where sequence or structural homology between human and Bb proteins cause B- and T-cell receptors to cross-react with an epitope on a Bb protein (the intended target) and a human protein (the unintended target). This could lead to ongoing inflammation of tissue and has been most well-studied in patients with LA ( 220 – 228 ). Four self-proteins (autoantigens) recognized by CD4 T cells have recently been identified in LD patients with LA through the use of tandem mass spectrometry on peptides in complex with MHC class II receptors (HLA-DR) ( 229 ). The four autoantigens identified include peptides from endothelial cell growth factor (ECGF), apolipoprotein B-100 (apoB-100), matrix metalloproteinase-10 (MMP-10), and annexin A2 proteins. Autoreactivity to these self-proteins appear to be primarily associated with LD, except annexin A2, which was associated with other rheumatic diseases ( 228 ), and potentially, to severe COVID-19 ( 230 ). To-date, no specific autoantigens have been identified reliably in LD. For the autoantigens that have been identified, we currently do not know whether the observed autoreactivity is induced by the presence of specific Bb antigens.
Persistence
Persistent antigenic debris.
Recent studies of Bb peptidoglycan have renewed interest in the potential roles this immunogenic macromolecule may play in LA and, more broadly, in disease pathogenesis. Peptidoglycan (PG) is an essential biopolymer that acts like a molecular bag—surrounding the bacterial cytoplasm and preventing cell bursting due to osmotic pressure. The cell walls of virtually all bacteria contain PG, and chemical and structural conservation is apparent [reviewed in ( 231 )]. Glycan strands, made up of the repeating disaccharide N -acetyl-glucosamine and N- acetylmuramic acid, are cross-linked by peptides composed of often alternating L and D-amino acids. Deviations from this chemical and conformational arrangement are rare, which make PG a quintessential pathogen-associated molecular pattern (PAMP) [reviewed in ( 232 , 233 )]. Recognition of bacterial peptidoglycans by innate immune system receptors [e.g., Toll-like receptors (TLRs), PG recognition proteins (PGRPs), and NOD proteins] leads to inflammation and the production of cytokines that can result in host tissue damage. Immune response(s) to bacterial PGs have been associated with symptoms of infections such as gonorrhea ( 234 , 235 ), chronic gastritis, and pertussis ( 236 ). There has been some evidence for a potential role for peptidoglycan in several autoimmune diseases, including rheumatoid arthritis ( 237 ) and multiple sclerosis ( 238 , 239 ).
The cell envelope of Bb contains a peptidoglycan (PG Bb ) that so far appears to be chemically unique, but conserved amongst some spirochetes. For example, close Borreliae relatives in the Relapsing Fever clade and Treponema genus have been reported to possess L-Orn-type PG ( 240 , 241 ). For bacteria to grow, divide, and ultimately cause disease, PG is continuously remodeled—small muropeptide fragments are removed and replaced with multimers. Unlike many other bacteria, Bb lacks the genetic components necessary for recycling the excised muropeptide fragments back into the cytoplasm. Instead, muropeptides are shed during growth and accumulate in logarithmic fashion that correlates with spirochete density ( 102 ). Analysis of radio-labeled, PG-associated amino acid over time, indicates that Bb sheds ~45% of its PG per generation ( 102 ). Despite the overall abundance PG shedding would cause, its role in LD pathogenesis remains to be fully elucidated.
PG Bb elicits an immune response in humans. This was first shown in 1990 when PG isolated from Bb was injected subcutaneously into the forearm of a volunteer, one of the co-authors of the report, who then experienced intense inflammation at the injection site for 72 h ( 242 ). Jutras et al. detected PG Bb in 94% of synovial fluid samples collected from 34 patients with LA ( 102 ). They also demonstrated the connection between PG Bb and disease pathogenesis through tail vein injection of PG Bb into mice and the subsequent observation of acute arthritis ( 102 ).
Much remains to be determined about the role of PG Bb in LA and, more broadly, in LD. For instance, are the PG remnants that of dead/dying bacteria following phagocytosis or antibiotic therapy or are they shed muropeptides? Transcript levels of Lysozyme—the human enzyme responsible for degrading PG—are elevated in LA patient SF ( 216 ), so why isn't it working or is the substrate absent (polymeric PG vs. muropeptides). The fate of shed muropeptide fragments, or their dwell time in different anatomical sites, is not known. In fact, the exact chemical composition of released muropeptide(s) is yet to be determined. Since released muropeptides must contain L-Orn ( 102 ), one intriguing possibility is that PG chemistry affects the response and/or half-life in the human host. Do germline variants or differential expression of human PGLYRP1 impact the pathogenesis of LD? Clearly, much remains to be elucidated, but methods toward preventing the human responses to PG Bb or eliminating the lingering antigen entirely, are two attractive avenues of future therapy in patients with persistent LD.
Persistent Infection
Like most bacteria, Bb are able to change their cellular phenotype in order to better survive in adverse conditions. Bb form persister cells, in vitro ( 85 , 243 ) or when exposed to antibiotics [( 243 ), bacterial “persistence' reviewed in ( 244 ), reviewed in ( 245 , 246 )]. The connection between persister cells, atypical morphological forms of Bb and disease pathogenesis in LD remains poorly understood [reviewed in ( 247 )].
Antibiotic resistance vs. antibiotic tolerance . Generally speaking, the ability of bacteria to grow in the presence of an antibiotic indicates resistance of a specific nature. Whether the antibiotic targets bacterial protein or nucleic acid synthesis, cell wall synthesis or integrity, or a specific metabolic pathway, uninhibited growth in the presence of that antibiotic demonstrates that the bacteria have acquired the ability to counteract that drug. Resistance may be inherent in the genome, acquired by horizontal gene transfer, or produced by mutation. Pseudomonas aeruginosa , for example, possesses multiple operons that encode efflux pumps ( 248 ) and Mycobacterium tuberculosis has become resistant by virtue of mutations which, for example, prevent the inhibitors of cell wall synthesis from binding to their target enzyme ( 249 , 250 ). Alternatively, bacteria may stunt their own replication in the presence of a bacteriostatic antibiotic, thus minimizing the effect. The latter is non-specific and can be referred to as antibiotic tolerance. Bb encodes an efflux pump system, but specific resistance to antibiotics has not been clearly demonstrated ( 251 ). The generation of slow-growing or non-growing “persister” cells in vitro is a well-established observation for multiple bacterial species ( 252 , 253 ). Bb , in particular, has been shown to tolerate multiple antibiotics ( 85 , 243 ) and form persisters by a stochastic mechanism leading to a slow-growing phenotype ( 254 ). Persister cells in vivo have not been demonstrated. In natural infection, the ability of bacteria to establish dormancy is perhaps best exemplified by Mycobacterium tuberculosis . Such infections can be latent for years and often never result in fulminant disease; the entry into dormancy is likely influenced by hypoxia or other environmental stressors ( 255 ). Another pathogenic spirochete, Treponema pallidum , can enter a chronic dormant phase within the human host and reactivate as tertiary syphilis years after the primary infection ( 256 ). How or where T. pallidum persists is not known.
If entry into a dormant phase occurs in vivo , the possibility for bacteria to tolerate growth-inhibiting compounds is a legitimate possibility. B. burgdorferi may enter dormancy during long periods of nutrient deprivation within the tick or following treatment of a mammalian host with a bacteriostatic antibiotic such as doxycycline. The stress response of the bacteria to nutrient deprivation has been described ( 257 ) and this capability to survive harsh environments may well contribute to antibiotic tolerance as well ( 258 ).
Bb infection, in particular . The question of the effectiveness of antibiotic treatment for LD has been contentious among physicians and researchers for some time ( 91 , 259 – 261 ). Among the challenges to determining if antimicrobial therapy is curative is the absence of reliable measures to determine that infection has been cleared from LD patients and the vague, non-specific symptoms with which patients present in PTLD ( 30 , 106 ). The notion that spirochetes may persist in humans derives primarily from the proportion of patients who experience symptoms post-treatment ( 107 , 262 ). A few studies have examined this phenomenon in humans—two in the U. S. [reviewed in ( 263 )] and one in the Netherlands ( 264 ). For both studies in the USA, only patients that had been treated for acute (early) LD were included and the authors concluded that resolution of symptoms did not occur with a subsequent 90-day treatment with antibiotics ( 265 ). While the Netherlands study also showed no significant difference between longer term treatment of patients with chronic symptoms and short-term therapy, the inclusion criteria were less stringent, potentially allowing patients without LD into the study. More recently, spirochetes were detected in post-mortem brain samples collected from a patient who previously was diagnosed and treated for LD and subsequently experienced chronic symptoms, including dementia ( 266 ).
Studies of persistence . To date, multiple studies in animals have shown that Bb spirochetes do persist following antibiotic treatment of a disseminated infection ( 267 – 269 ). Even in humans, rare evidence of possible persistence was gleaned from feeding uninfected ticks on a patient with PTLD ( 270 ). In this study, only Bb DNA was detected while attempts to visualize or culture viable spirochetes from the xenodiagnostic ticks were unsuccessful. As others have noted, without the recovery of metabolically active spirochetes, this experiment is suggestive but is not a clear demonstration of persistent infection in humans ( 271 ).
Evidence from experiments performed in mice and dogs reveals that spirochetes can persist in the mammalian host after the administration of antimicrobial drugs. In one study, dogs were treated with a 30-day course of amoxicillin or doxycycline 2 months after infection ( 267 ). Spirochetes were recovered from tissues in 3 of 12 dogs. Skin punch biopsy samples from nearly all dogs were PCR-positive for Bb after treatment. Interestingly, serum antibodies to Bb declined post-treatment, but after the dogs were kept 6 months in pathogen-free housing, their antibody titers rose, indicating recrudescence. Spirochetal persistence has been examined in mice using xenodiagnostic studies in which naïve ticks were placed on infected mice that received a course of antibiotics ( 272 – 274 ). Those ticks acquired spirochetes from the mice post-treatment, detected by fluorescent imaging ( 272 ) or PCR ( 273 , 274 ). Two of these studies examined the existence of persistent spirochetes as a function of the time elapsed prior to treatment ( 273 , 274 ). Spirochetal DNA was more frequently detected in xenodiagnostic ticks (XT) that fed upon mice treated 4 months post-infection than from those treated 3 weeks post-infection. When XT that had acquired organisms from antibiotic-treated mice fed upon naïve mice, the mice harbored spirochetal DNA in multiple tissues (detected by PCR), but organisms could not be recovered by tissue culture. Finally, studies in primates have shown that morphologically intact spirochetes can persist following antibiotic treatment ( 268 ). In a subsequent study, not only were the spirochetes found intact after treatment, but were shown to be transcriptionally active and were detected in multiple tissues, including the brain and heart ( 275 , 276 ). This is evidence to suggest that the organisms are not fully cleared and may be attenuated for infection or for recovery by tissue culture.
With rare exception ( 127 ), only Bb genetic material (DNA or RNA), antigen, or non-culturable spirochetes have been detected following antibiotic treatment of an established infection. In none of the aforementioned animal studies has the pathogen been recovered as indicated by spirochete replication in culture soon (1–2 weeks) after inoculation of the standard BSK medium with tissue or tick specimen. Some experts in the field have therefore surmised that the spirochetes are non-viable and therefore that the infection is not persistent ( 103 , 271 , 277 , 278 ). Evidence of resurgence in mice that were evaluated a year after antibiotic treatment contradicts this notion of non-viability ( 269 ). In that study, the amount of Bb genetic material in each mouse was quantified and found to increase from a very low level a few months after treatment to levels as high as in untreated animals at 12 months after treatment, indicating that the spirochetes replicated. The Bb bacteria present after antibiotic treatment, which are metabolically active and appear to be capable of resurging in vivo or resuscitated under the right culture conditions have been deemed “viable, but non-culturable (VBNC).”
The VBNC state is not unique to Bb , but rather, it is known to occur in over 100 other bacterial species studied to date ( 279 ). Entering dormancy of this “nonculturable” type is very common for bacterial pathogens ( 280 , 281 ). The VBNC state has been characterized as a deeper state of dormancy than that of persister cells, observed in several Vibrio species, E. coli, Campylobacter, Burkholderia, Listeria, Salmonella , and Helicobacter ( 281 – 283 ).
Persistent infection with Bb is difficult to rule-in or out as an explanation for LD patients with ongoing symptoms due to the challenge of culturing viable spirochetes from human specimens except in the earliest stages of infection, prior to antibiotic treatment ( 21 ). The failure to reliably isolate metabolically active spirochetes from patients does not exclude the possibility that they exist in some patients with ongoing health problems.
Ecological Prevention
In the USA, Bb is the most common vector-borne pathogen; LD comprises 62.6% of all vector-borne diseases and 81.2% of all tick-borne diseases ( 1 ). There is an increasing trend of new cases in counties and states neighboring high-incidence regions, indicating a spread of the pathogen and disease risk in new geographical areas ( 52 ). The current complexities around the diagnosis and treatment of LD and PTLD suggest a growing need for primary prevention and to understand the intricacies of the ecological factors that impact disease risk.
LD risk, and the broader goal of prevention of LD, is commonly viewed through two lenses: an ecological approach that focuses on characteristics of the tick vector, its hosts and the pathogens it transmits; and a human behavior approach that examines how behaviors and attitudes of human individuals, such as frequency of outdoor activities or use of protective equipment, change risk to disease ( 284 , 285 ). Indirect factors regarding host populations, abiotic conditions, and land use or land coverage have also been found to increase disease risk, yet the magnitude of impact or relation to tick encounters and infection risk is still not fully understood ( 284 , 286 ).
Popular ecological preventative techniques gravitate around reduction of host populations, reduction of ticks, and reduction of pathogen infection in ticks or hosts. Popular human-behavioral strategies include altering the risk of exposure of humans through behavioral changes associated with self-protection, use of outdoor space, and modifications of the environment. White-footed mice are the primary reservoir for Bb and their density has been shown to affect LD risk ( 287 – 290 ). The culling of white-tailed deer is a common preventative technique, but research sheds doubt on the viability or practicality of mass culling, suggesting that the technique is only effective on islands or closed populations where complete elimination can be accomplished ( 291 ). Personal protective measures, including checking body for ticks and use of tick repellent are frequently promoted by government and public health agencies. Some of these measures have been shown to reduce disease risk, yet effectiveness may be as low as 20–40%, with some practices like checking one's body for ticks being found ineffective ( 284 , 292 , 293 ). One challenge to prevention is the fact that nymphal ticks are as small as a poppy seed, and their bites can easily go unnoticed. Land usage or cover has shown strong trends of being impactful on exposure to LD, but still being researched is the spatial scale of land usage ( 284 ). Questions of land use in residential spaces or neighborhoods are still being explored, as well as the human movement within those spaces ( 294 ).
Several research projects have been initiated to further explore LD prevention by minimizing infection risk. The Tick Project is undertaking an immense randomized control trial of effectiveness of Met52 fungal spray and Tick Control System rodent bait boxes for LD prevention across 24 neighborhoods in Dutchess County, New York, while also collecting and assessing data on the entomological and host population risk factors, tracking tick encounters, and documenting cases of LD and PTLD across these neighborhoods ( 295 ). New models are being created to predict the first incidences of LD in counties without any reported cases of LD based on abiotic and human behavior factors ( 296 ). Prediction models will need to account for climate change as a contributing factor to the expanding range of LD. In addition, there are still several gaps in our knowledge about effective preventative techniques that should be further studied: abiotic factors and the capability of predictive modeling, diversity of Bb strains in tick and host populations and its impact on disease risk, predator communities and their role on host communities, changing landscapes and urban spaces, and the costs, sustainability, and acceptability to the public of preventative techniques ( 286 , 288 , 290 , 297 ).
Human Vaccine
There is an urgent need for a safe and effective human vaccine that targets multiple pathogenic Borreliella species and strains, or even more broadly, common co-infections. Currently, there is no human vaccine for LD commercially available in the USA. In 1998, the FDA licensed Lymerix, a recombinant Osp-A based vaccine for the prevention of LD in adults. The vaccine required three shots over two tick seasons and was reported to be 76% effective in the prevention of LD after the third shot ( 49 ). The mechanism of action of this and other Osp-A based vaccines is antibody-mediated blocking of the transmission of Borreliella spirochetes from infected ticks while feeding on a human host ( 298 , 299 ). Public demand and acceptance of the vaccine was low for a variety of factors reviewed elsewhere ( 300 – 304 ) and Lymerix was pulled from the market by its manufacturer, Smith-Kline Beecham in 2002. Interest from industry waned and other efforts to develop Lyme vaccine candidates were also abandoned, including those by MedImmune, Baxter and Connaught Laboratories ( 300 ).
Twenty years after Lymerix, there are now multiple efforts underway to develop next-generation human vaccines for the prevention of LD. One challenge that these vaccines must address is the genetic diversity of pathogenic Borreliella species and strains across different geographies. Even a single infected tick may carry multiple heterologous strains of Bb. Therefore, current vaccine candidates incorporate multiple immunogenic antigens or multiple serotypes of a single immunogenic antigen. Outer surface proteins, especially OspA-C, are the most common antigens selected among current human vaccine candidates, but other antigens and vaccine strategies are being studied [reviewed in ( 305 )].
VLA15 is currently the only Lyme vaccine candidate in human trials. VLA15 uses recombinant outer surface protein A (OspA) from six different OspA serotypes of pathogenic Borreliella species, including Bb (OspA serotype 1), B. afzelii (OspA serotype 2), B. garinii (OspA serotypes 3, 5, 6), and B. bavariensis (OspA serotype 4) ( 306 – 308 ). In a similar approach, a prototype vaccine that uses bacterial ferritin nanoparticles fused with seven serotypes of OspA molecules from Bb, B. afzelii, B. bavariensis and B. garinii recently showed durable high-titer antibody response in both mouse and rhesus macaque animal models ( 309 ). In both vaccine candidates, the antigenic residues in Osp-A serotype 1 that were previously suspected ( 221 ) but never shown to be cross-reactive were removed ( 304 , 309 ).
There are several promising strategies for the development of other novel human vaccines for LD in experimental and preclinical stages. Marconi et al. has conducted studies in dogs on a subunit vaccine that includes OspA and at least 14 immunogenic linear epitopes (“chimeritope”) from diverse isotypes of OspC. These outer surface proteins are expressed while Bb is in the tick gut (OspA) or during early human infection (OspC) [( 305 , 310 – 312 ), reviewed in ( 313 )]. The OspC chimeritope is a component of the most widely used Lyme vaccine for dogs ( 314 ). By combining antigens that are expressed by Borreliella at different stages of infection, this vaccine has the potential to protect against spirochetes that are not blocked or killed while in the tick gut. This vaccine has not yet entered human trials. Another experimental vaccine targets Cspz, an outer surface protein involved in complement evasion ( 315 ).
The development of an anti-tick vaccine is one potential approach to protect people from multiple tick-borne diseases, including LD, as recently reviewed elsewhere ( 316 ). Ixodes scapularis ticks transmit 16 human pathogens ( 317 ) associated with tick-borne disease in the USA, including Bb, Borrelia miyamotoi ( 318 , 319 ), Babesia microti ( 320 , 321 ), Anaplasma phagocytophilum ( 320 , 322 ), Ehrlichia muris-like agent (EMLA) ( 323 ) and Powassan virus ( 324 , 325 ). During transmission to a human, bacteria interact with tick proteins in the gut and salivary glands. These interactions can influence whether transmission occurs. Increased protection might be conferred if any of several steps in the transmission cycle are inhibited by targeting one or several of these tick proteins simultaneously. For example, mice that were given antiserum to the tick protein, Salp15, and then were challenged with Bb , showed protection from colonization ( 326 ). Tick proteins may also elicit “tick immunity,” a process during which a host becomes resistant to tick bites because the ticks cannot feed properly ( 327 ). If a vaccine can be developed that creates tick immunity in humans, this may enable the prevention of LD, and other tick-borne diseases, especially for those that migrate slowly from tick to human ( 305 , 328 – 332 ). Viruses, such as Powassan, can be transmitted from tick to the human host in mere minutes. The attachment time required for tick-borne pathogens to migrate from tick to host was recently reviewed elsewhere ( 333 ). Encouragingly, there is already one commercially available tick vaccine used for the protection of livestock against tick infestation, though not including Ixodes spp . ticks ( 334 – 336 ).
Field Building
To improve our ability to better address Lyme and other tick-borne diseases, we need to attract researchers to the field and build shared resources that accelerate research progress such as biorepositories, genomic resources, animal models, and preclinical services ( 57 ). Interest in LD research over the past 5 years has remained relatively flat: the term “Lyme disease” is mentioned in around ~1,000 publications per year combined from PubMed Central in the USA and Europe. In this section, we describe several key resources for investigators seeking to study LD.
Biorepositories and Research Cohorts
Well-characterized samples are an essential tool to help researchers develop and validate new diagnostic tests and to better understand the complexities of LD. Well-characterized sample sets can benefit medical providers, test developers, and the public at risk for LD ( 337 ). It is critical that sample users understand the criteria used to enroll participants, how samples were collected and stored, and what additional clinical and testing data may be available. Additional benefits can be realized when multiple sample users (test developers and researchers) are using the same well-characterized sample sets. Current sample sets available for researchers include the CDC Lyme Serum Repository (LSR) ( 337 ), the Lyme Disease Biobank ( 23 ), and samples from the Studies of Lyme Immunology and Clinical Events (SLICE) at Johns Hopkins University School of Medicine. Additionally, some investigators also have their own sample collections with, in some cases, blood samples, skin biopsy specimens and synovial fluid which form the basis for collaborative studies ( 69 , 102 , 338 ).
Lyme Disease Biobank
The Lyme Disease Biobank is a collection of more than 900 human biological samples that facilitates research in the field of LD and other tick-borne infections (TBI). Whole blood, serum and urine samples are collected from individuals presenting with the signs and symptoms of early LD with or without an EM lesion, individuals with later stages of LD including persistent LD, and unaffected individuals (endemic controls). Samples have been collected from the East Coast, Upper Midwest, and California. Robust clinical information accompanies the samples, including information about symptoms, EM (if present), current medications, history of LD and other TBI, medical history, and demographics. Photographs of EM lesions are also taken (if present). Participants enrolled with early LD also have the option of providing a convalescent sample 2–3 months after the initial blood draw. PCR testing is performed, in a blinded fashion, to confirm the presence of Bb, Anaplasma phagocytophilum, Babesia microti , and B. miyamotoi in the samples. Serologic assays for standard two-tiered testing analysis (ELISA followed by IgM/ IgG Western immunoblotting) have also been performed. Each participant's donation provides samples for ~50 research projects, with aliquots of whole blood (1 and 2 ml), serum (250 μl), and urine (1 ml) available to sample users in academia and industry. More than 10,000 aliquots have been distributed across 50+ diagnostic research projects. The characterization of samples from 550 participants enrolled on the East Coast and Upper Midwest is detailed here ( 23 ). Specific panels are also available, including an unblinded panel for projects earlier in development, panels of later stages of LD including Lyme carditis and neuroborreliosis, panels of other TBI, as well as samples from patients with persistent LD. A tissue bank was recently launched for post-mortem and surgical samples. Tissue donors have the option to link their MyLymeData registry profile to their tissue sample ( 339 , 340 ).
Lyme Disease Research Center
The primary focus of the Johns Hopkins Lyme Disease Research Center (LDRC) is clinical translational research to advance the fundamental understanding of LD through the characterization of carefully constructed cohorts of LD patients and controls, as well as a clinical biorepository of blood and tissue biospecimens. The LDRC enrolls participants from an expanded Mid-Atlantic region into a variety of research protocols, which all collect detailed health histories, clinical, and behavioral data. Over 350 participants have been enrolled in ongoing longitudinal cohort studies (some followed for up to 10 years), which include patients meeting CDC criteria for early and late LD, as well as uninfected controls without LD. An additional 275 participants meeting the IDSA definition for PTLD have also been enrolled in a clinical case series study ( 44 ). The SLICE studies obtain a number of different biosamples including: a skin biopsy (in patients with acute LD), whole blood, serum, plasma, PBMCs, DNA, RNA, skin and fecal swabs, and most recently, urine. All these samples are processed in the laboratory, aliquoted, archived and stored. To-date, they have shared ~6,000 sample aliquots for collaborative initiatives. The center collaborates with key investigators who utilize these samples for immune profiling ( 209 ), transcriptomics ( 67 , 68 ), proteomics ( 69 ), metabolomic ( 75 ), and microbiome-based studies ( 58 ). These studies generate rich and deep clinical and molecular data sets that may allow for new insights into LD pathophysiology, lead to new diagnostic and therapeutic approaches, and have contributed to the characterization of LD and PTLD longitudinally across dozens of clinical and neurobehavioral variables. The LDRC has demonstrated that PTLD is a definable condition that is distinguishable from those that “return to health” following infection and treatment for LD. With the ability to compare PTLD patients with controls uninfected with LD, the SLICE studies show that the rates of individuals with both symptoms and a decline in health-related quality of life are significantly higher in patients previously treated for acute LD than in controls (14 vs. 4%) (unpublished data). The growth of these cohorts over the years is also evidence that it is possible to not only recruit patients with PTLD into research, but also maintain their participation at high levels.
Long Island Outdoor Worker Cohort
To investigate the seasonal incidence and seroprevalence rates, a team of investigators at the Stony Brook School of Medicine assessed outdoor workers in the Hispanic/Latino immigrant population residing in Eastern Long Island and compared rates to those of non-immigrant outdoor workers. To further investigate occupational risk, they looked at differences of incidence rates in field workers and non-field workers within the Hispanic/Latino immigrant population. The study shows a significantly higher rate of Bb exposure among Hispanic/Latino immigrant field workers compared to those belonging to other occupations and in non-immigrant outdoor workers and also sheds light on the epidemiology, seroprevalence, and seasonal incidence of Lyme and other tick-borne diseases, as well as their clinical manifestations. Treatment and prevention of LD in this population can be especially difficult to obtain when multiple barriers are in place, such as poor health literacy, lack of preventative measures and limited access to healthcare in those with more risk. These findings underscore the necessity of improved education and preventative measures to better protect this vulnerable population ( 341 , 342 ).
Data Repositories
Lymemind commons.
The LymeMIND center at the Icahn School of Medicine at Mount Sinai is developing LymeMIND Commons ( https://commons.lymemind.org/ ), an online database and a search engine that contains collected data and metadata integrated from the consortium and other LD resources. LymeMIND Commons enables researchers with the ability to find and analyze various types of data and metadata related to LD. Beyond transcriptomics, data includes protein arrays, methylation profiling by high throughput sequencing, genotyping, and other platforms. The metadata in LymeMIND Commons is JSON serialized and hosted using the Signature Commons platform. The metadata within LymeMIND Commons is linked to external ontologies and other resources for each study. In summary, LymeMIND Commons serves as a unique resource to advance LD research.
More research into the prevention, diagnosis and treatment of LD is needed in order to address the significant health risks posed by this tick-borne disease. The annual NIH and CDC investment in Lyme and tick-borne diseases research has been relatively unchanged for decades and is small compared to many other infectious diseases. In 2015, the Steven & Alexandra Cohen Foundation established a research consortium involving over 30 leading universities, research laboratories and other organizations that aim to advance Lyme and tick-borne disease diagnosis and treatment, human vaccination, awareness and education, data science and management, and ecological prevention. Philanthropic funding, including a new public-private partnership around novel diagnostic technologies, is critical to address the historically small amount of federal funding for LD compared to some other infectious disease of public health concern. While the Kay Hagan Tick Act did recently boost federal support for LD research ( 343 ), more is needed.
LD is a large topic. Some important advances were excluded for space considerations, such as immune evasion by Bb spirochetes, common co-infections, model organisms and in vitro systems for the study of LD, neuronal sensitization hypothesis for the etiology of PTLD specifically and neuroborreliosis in general, among others.
In closing, in consideration of the unique global circumstances with the COVID-19 pandemic, it is important to highlight several features of the current context that may impact the LD community. For example, COVID-19 may further complicate diagnosis of LD since non-specific symptoms in these two conditions overlap and people may be spending more time outdoors. The emergence of a persistent syndrome, popularly referred to as long COVID, among a subset of patients following treatment or convalescence ( 344 ) may invigorate research and provide insights that carry-over into other infectious diseases with post-treatment sequelae, such as LD.
Author Contributions
JRB wrote the first draft of the manuscript. BLJ, EJH, MEE, AB, and RLM wrote sections of the manuscript. All authors contributed to manuscript revision, read, and approved the submitted version.
All authors are supported by funding from the Steven & Alexandra Cohen Foundation. Additional funding is provided by grants from the Global Lyme Alliance and NIH/NIAID R01AI157007 (NB); Bay Area Lyme Foundation (JRB); NIH R01 AI083640 (FY); NIAID/NIH R21AI133335 and R21AI142302 (RLM and PJR).
Conflict of Interest
AGR is a scientific advisor to NeonMind Biosciences and ETHA Natural Botanicals. In conjunction with Dr. S. Sontakke and North Carolina State University, EBB holds US Patent No. 7,115,385; Media and Methods for Cultivation of Microorganisms, which was issued on October 3rd, 2006. He is a co-founder, shareholder and Chief Scientific Officer for Galaxy Diagnostics, a company that provides advanced diagnostic testing for the detection of Bartonella spp. and Borrelia infections. RM is a co-founder, shareholder and the Chief Technical Officer for Galaxy Diagnostics. RTM is a paid consultant/speaker for Zoetis and receives license related income from Zoetis. MJS serves on the Scientific Advisory Board for the Global Lyme Alliance and has been issued a patent [US Patent No. 10 481 165] for “Elevated CCL19 after completion of therapy for acute Lyme disease identifies patients at risk for development of post-treatment Lyme disease syndrome who will benefit from further antibiotic therapy”. AO has pending patent applications on the development of Lyme disease diagnostic tests.
The remaining authors declare that the research was conducted in the absence of any commercial or financial relationships that could be construed as a potential conflict of interest.
Publisher's Note
All claims expressed in this article are solely those of the authors and do not necessarily represent those of their affiliated organizations, or those of the publisher, the editors and the reviewers. Any product that may be evaluated in this article, or claim that may be made by its manufacturer, is not guaranteed or endorsed by the publisher.
1. ^ https://www.columbia-lyme.org/clinical-trials-network
1. Rosenberg R, Lindsey NP, Fischer M, Gregory CJ, Hinckley AF, Mead PS, et al. Vital signs: trends in reported vectorborne disease cases - United States and Territories, 2004-2016. MMWR Morb Mortal Wkly Rep. (2018) 67:496–501. doi: 10.15585/mmwr.mm6717e1
PubMed Abstract | CrossRef Full Text | Google Scholar
2. Kugeler K, Schwartz A, Delorey M, Mead P, Hinckley A. Estimating the frequency of Lyme disease diagnoses, United States, 2010–2018. Emerg Infect Dis J. (2021) 27:616–9. doi: 10.3201/eid2702.202731
3. Nelson CA, Saha S, Kugeler KJ, Delorey MJ, Shankar MB, Hinckley AF, et al. Incidence of clinician-diagnosed Lyme disease, United States, 2005-2010. Emerg Infect Dis. (2015) 21:1625–31. doi: 10.3201/eid2109.150417
4. Steere AC, Strle F, Wormser GP, Hu LT, Branda JA, Hovius JWR, et al. Lyme borreliosis. Nat Rev Dis Primers. (2016) 2:16090. doi: 10.1038/nrdp.2016.90
5. Adrion ER, Aucott J, Lemke KW, Weiner JP. Health care costs, utilization and patterns of care following Lyme disease. PLoS ONE. (2015) 10:e0116767. doi: 10.1371/journal.pone.0116767
6. TBDWG. Tick-Borne Disease Working Group: Report to Congress 2018 . HHS (2018). Available online at: https://www.hhs.gov/sites/default/files/tbdwg-report-to-congress-2018.pdf (accessed July 22, 2021).
7. Moon KA, Pollak J, Poulsen MN, Hirsch AG, DeWalle J, Heaney CD, et al. Peridomestic and community-wide landscape risk factors for Lyme disease across a range of community contexts in Pennsylvania. Environ Res. (2019) 178:108649. doi: 10.1016/j.envres.2019.108649
8. Nigrovic LE, Bennett JE, Balamuth F, Levas MN, Chenard RL, Maulden AB, et al. Accuracy of clinician suspicion of Lyme disease in the emergency department. Pediatrics. (2017) 140:e20171975. doi: 10.1542/peds.2017-1975
9. Burlina PM, Joshi NJ, Mathew PA, Paul W, Rebman AW, Aucott JN. AI-based detection of erythema migrans and disambiguation against other skin lesions. Comput Biol Med. (2020) 125:103977. doi: 10.1016/j.compbiomed.2020.103977
10. Mead PS. Epidemiology of Lyme disease. Infect Dis Clin North Am. (2015) 29:187–210. doi: 10.1016/j.idc.2015.02.010
11. Hirsch AG, Poulsen MN, Nordberg C, Moon KA, Rebman AW, Aucott JN, et al. Risk factors and outcomes of treatment delays in Lyme disease: a population-based retrospective cohort study. Front Med. (2020) 7:560018. doi: 10.3389/fmed.2020.560018
12. Hirsch AG, Herman RJ, Rebman A, Moon KA, Aucott J, Heaney C, et al. Obstacles to diagnosis and treatment of Lyme disease in the USA: a qualitative study. BMJ Open. (2018) 8:e021367. doi: 10.1136/bmjopen-2017-021367
13. Rebman AW, Bechtold KT, Yang T, Mihm EA, Soloski MJ, Novak CB, et al. The clinical, symptom, and quality-of-life characterization of a well-defined group of patients with posttreatment Lyme disease syndrome. Front Med. (2017) 4:224. doi: 10.3389/fmed.2017.00224
14. Johnson L, Aylward A, Stricker RB. Healthcare access and burden of care for patients with Lyme disease: a large United States survey. Health Policy. (2011) 102:64–71. doi: 10.1016/j.healthpol.2011.05.007
15. Tibbles CD, Edlow JA. Does this patient have erythema migrans? JAMA. (2007) 297:2617–27. doi: 10.1001/jama.297.23.2617
16. Diuk-Wasser MA, Hoen AG, Cislo P, Brinkerhoff R, Hamer SA, Rowland M, et al. Human risk of infection with Borrelia burgdorferi, the Lyme disease agent, in eastern United States. Am J Trop Med Hyg. (2012) 86:320–7. doi: 10.4269/ajtmh.2012.11-0395
17. Nieto NC, Porter WT, Wachara JC, Lowrey TJ, Martin L, Motyka PJ, et al. Using citizen science to describe the prevalence and distribution of tick bite and exposure to tick-borne diseases in the United States. PLoS ONE. (2018) 13:e0199644. doi: 10.1371/journal.pone.0199644
18. California Department of Public Health Vector-Borne Disease Section. Epidemiology and Prevention of Tick-Borne Diseases in California . Available online at: https://westnile.ca.gov/pdfs/EpidemiologyandPreventionofTBDinCA.pdf (accessed July 7, 2021).
19. Centers for Disease Control and Prevention (CDC). Recommendations for test performance and interpretation from the Second National Conference on Serologic Diagnosis of Lyme Disease. MMWR Morb Mortal Wkly Rep . (1995) 44: 590–1.
Google Scholar
20. Mead P, Petersen J, Hinckley A. Updated CDC recommendation for serologic diagnosis of Lyme disease. MMWR Morb Mortal Wkly Rep. (2019) 68:703. doi: 10.15585/mmwr.mm6832a4
21. Aguero-Rosenfeld ME, Wang G, Schwartz I, Wormser GP. Diagnosis of lyme borreliosis. Clin Microbiol Rev. (2005) 18:484–509. doi: 10.1128/CMR.18.3.484-509.2005
22. Theel ES. The past, present, and (possible) future of serologic testing for Lyme disease. J Clin Microbiol. (2016) 54:1191–6. doi: 10.1128/JCM.03394-15
23. Horn EJ, Dempsey G, Schotthoefer AM, Prisco UL, McArdle M, Gervasi SS, et al. The Lyme disease biobank - characterization of 550 patient and control samples from the east coast and upper midwest of the United States. J Clin Microbiol . (2020) 58:26. doi: 10.1128/JCM.00032-20
24. Mosel MR, Rebman AW, Carolan HE, Montenegro T, Lovari R, Schutzer SE, et al. Molecular microbiological and immune characterization of a cohort of patients diagnosed with early Lyme disease. J Clin Microbiol . (2020) 59:e00615–20. doi: 10.1128/JCM.00615-20
25. Branda JA, Linskey K, Kim YA, Steere AC, Ferraro MJ. Two-tiered antibody testing for Lyme disease with use of 2 enzyme immunoassays, a whole-cell sonicate enzyme immunoassay followed by a VlsE C6 peptide enzyme immunoassay. Clin Infect Dis. (2011) 53:541–7. doi: 10.1093/cid/cir464
26. Branda JA, Steere AC. Laboratory diagnosis of Lyme borreliosis. Clin Microbiol Rev. (2021) 34:e00018–19. doi: 10.1128/CMR.00018-19
27. Wormser GP, Nowakowski J, Nadelman RB, Visintainer P, Levin A, Aguero-Rosenfeld ME. Impact of clinical variables on Borrelia burgdorferi -specific antibody seropositivity in acute-phase sera from patients in North America with culture-confirmed early Lyme disease. Clin Vaccine Immunol. (2008) 15:1519–22. doi: 10.1128/CVI.00109-08
28. Steere AC, McHugh G, Damle N, Sikand VK. Prospective study of serologic tests for lyme disease. Clin Infect Dis. (2008) 47:188–95. doi: 10.1086/589242
29. Rebman AW, Crowder LA, Kirkpatrick A, Aucott JN. Characteristics of seroconversion and implications for diagnosis of post-treatment Lyme disease syndrome: acute and convalescent serology among a prospective cohort of early Lyme disease patients. Clin Rheumatol. (2015) 34:585–9. doi: 10.1007/s10067-014-2706-z
30. Rebman AW, Aucott JN. Post-treatment lyme disease as a model for persistent symptoms in Lyme disease. Front Med. (2020) 7:57. doi: 10.3389/fmed.2020.00057
31. Strobino B, Steinhagen K, Meyer W, Scheper T, Saschenbrecker S, Schlumberger W, et al. A community study of Borrelia burgdorferi antibodies among individuals with prior Lyme disease in endemic areas. Healthcare. (2018) 6:69. doi: 10.3390/healthcare6020069
32. Aguero-Rosenfeld ME. Lyme disease: laboratory issues. Infect Dis Clin North Am. (2008) 22:301–13, vii. doi: 10.1016/j.idc.2007.12.005
33. Seriburi V, Ndukwe N, Chang Z, Cox ME, Wormser GP. High frequency of false positive IgM immunoblots for Borrelia burgdorferi in clinical practice. Clin Microbiol Infect. (2012) 18:1236–40. doi: 10.1111/j.1469-0691.2011.03749.x
34. Eldin C, Raffetin A, Bouiller K, Hansmann Y, Roblot F, Raoult D, et al. Review of European and American guidelines for the diagnosis of Lyme borreliosis. Med Mal Infect. (2019) 49:121–32. doi: 10.1016/j.medmal.2018.11.011
35. Sanchez JL. Clinical manifestations and treatment of Lyme disease. Clin Lab Med. (2015) 35:765–78. doi: 10.1016/j.cll.2015.08.004
36. Pachner AR. Neurologic manifestations of lyme disease, the new “great imitator.” Rev Infect Dis . (1989) 11:S1482–6. doi: 10.1093/clinids/11.Supplement_6.S1482
37. Fallon BA, Sotsky J, Brenner C, Makous M. Conquering Lyme Disease : Science Bridges the Great Divide with Columbia University Press . (2017). Available online at: https://books.google.com/books/about/Conquering_Lyme_Disease.html?id=-Ds9DwAAQBAJ (accessed July 22, 2021).
38. Yeung C, Baranchuk A. Diagnosis and treatment of Lyme carditis: JACC review topic of the week. J Am Coll Cardiol. (2019) 73:717–26. doi: 10.1016/j.jacc.2018.11.035
39. Steere AC, Sikand VK, Schoen RT, Nowakowski J. Asymptomatic infection with Borrelia burgdorferi . Clin Infect Dis . (2003) 37:528–32. doi: 10.1086/376914
40. Sigal LH. Toward a more complete appreciation of the clinical spectrum of Borrelia burgdorferi infection: early Lyme disease without erythema migrans. Am J Med. (2003) 114:74–5. doi: 10.1016/S0002-9343(02)01439-0
41. Carlsson H, Sandholm K, Haddish HW, Brudin L, Ekdahl KN, Tjernberg I. Complement activation in individuals with previous subclinical Lyme borreliosis and patients with previous Lyme neuroborreliosis. Eur J Clin Microbiol Infect Dis. (2019) 39:855–62. doi: 10.1007/s10096-019-03807-5
42. Kugeler KJ, Griffith KS, Gould LH, Kochanek K, Delorey MJ, Biggerstaff BJ, et al. A review of death certificates listing Lyme disease as a cause of death in the United States. Clin Infect Dis. (2011) 52:364–7. doi: 10.1093/cid/ciq157
43. Semproni M, Rusk R, Wuerz T. Fatal Lyme carditis presenting as fluctuating high-grade atrioventricular block. CMAJ. (2020) 192:E574–7. doi: 10.1503/cmaj.191194
44. Wormser GP, Dattwyler RJ, Shapiro ED, Halperin JJ, Steere AC, Klempner MS, et al. The clinical assessment, treatment, and prevention of Lyme disease, human granulocytic anaplasmosis, and babesiosis: Clinical Practice Guidelines by the Infectious Diseases Society of America. Clin Infect Dis. (2006) 43:1089–134. doi: 10.1086/508667
45. Feder HM Jr, Whitaker DL. Misdiagnosis of erythema migrans. Am J Med. (1995) 99:412–9. doi: 10.1016/S0002-9343(99)80190-9
46. Aucott JN, Crowder LA, Yedlin V, Kortte KB. Bull's-eye and nontarget skin lesions of Lyme disease: an internet survey of identification of erythema migrans. Dermatol Res Pract. (2012) 2012:451727. doi: 10.1155/2012/451727
47. Schutzer SE, Berger BW, Krueger JG, Eshoo MW, Ecker DJ, Aucott JN. Atypical erythema migrans in patients with PCR-positive lyme disease. Emerg Infect Dis J. (2013) 19:815. doi: 10.3201/eid1905.120796
48. Dennison R, Novak C, Rebman A, Venkatesan A, Aucott J. Lyme disease with erythema migrans and seventh nerve palsy in an African-American Man. Cureus. (2019) 11:e6509. doi: 10.7759/cureus.6509
49. Steere AC, Sikand VK, Meurice F, Parenti DL, Fikrig E, Schoen RT, et al. Vaccination against Lyme disease with recombinant Borrelia burgdorferi outer-surface lipoprotein a with adjuvant. N Engl J Med. (1998) 339:209–15. doi: 10.1056/NEJM199807233390401
50. Steere AC, Dhar A, Hernandez J, Fischer PA, Sikand VK, Schoen RT, et al. Systemic symptoms without erythema migrans as the presenting picture of early Lyme disease. Am J Med. (2003) 114:58–62. doi: 10.1016/S0002-9343(02)01440-7
51. Aucott J, Morrison C, Munoz B, Rowe PC, Schwarzwalder A, West SK. Diagnostic challenges of early Lyme disease: lessons from a community case series. BMC Infect Dis. (2009) 9:79. doi: 10.1186/1471-2334-9-79
52. Schwartz AM, Hinckley AF, Mead PS, Hook SA, Kugeler KJ. Surveillance for Lyme Disease - United States, 2008-2015. MMWR Surveill Summ. (2017) 66:1–12. doi: 10.15585/mmwr.ss6622a1
53. Lagier J-C, Edouard S, Pagnier I, Mediannikov O, Drancourt M, Raoult D. Current and past strategies for bacterial culture in clinical microbiology. Clin Microbiol Rev. (2015) 28:208–36. doi: 10.1128/CMR.00110-14
54. Franco-Duarte R, Cernáková L, Kadam S, Kaushik KS, Salehi B, Bevilacqua A, et al. Advances in chemical and biological methods to identify microorganisms-from past to present. Microorganisms. (2019) 7:130. doi: 10.3390/microorganisms7050130
55. Wormser GP. Hematogenous dissemination in early Lyme disease. Wien Klin Wochenschr. (2006) 118:634–7. doi: 10.1007/s00508-006-0688-9
56. Nowakowski J, McKenna D, Nadelman RB, Bittker S, Cooper D, Pavia C, et al. Blood cultures for patients with extracutaneous manifestations of Lyme disease in the United States. Clin Infect Dis. (2009) 49:1733–5. doi: 10.1086/648076
57. NIH Strategic Research Plan Addresses Growing Tickborne Diseases Threat . National Institutes of Health (NIH) (2019). Available online at: https://www.nih.gov/news-events/news-releases/nih-strategic-research-plan-addresses-growing-tickborne-diseases-threat (accessed January 14, 2020).
58. Morrissette M, Pitt N, González A, Strandwitz P, Caboni M, Rebman AW, et al. A distinct microbiome signature in posttreatment Lyme disease patients. MBio. (2020) 11:e02310–20. doi: 10.1128/mBio.02310-20
59. Fitzgerald BL, Graham B, Delorey MJ, Pegalajar-Jurado A, Islam MN, Wormser GP, et al. Metabolic response in patients with post-treatment Lyme disease symptoms/syndrome. Clin Infect Dis . (2020). doi: 10.1093/cid/ciaa1455. [Epub ahead of print].
60. Tokarz R, Mishra N, Tagliafierro T, Sameroff S, Caciula A, Chauhan L, et al. A multiplex serologic platform for diagnosis of tick-borne diseases. Sci Rep. (2018) 8:3158. doi: 10.1038/s41598-018-21349-2
61. Arumugam S, Nayak S, Williams T, di Santa Maria FS, Guedes MS, Chaves RC, et al. A multiplexed serologic test for diagnosis of Lyme disease for point-of-care use. J Clin Microbiol. (2019) 57:e01142–19. doi: 10.1128/JCM.01142-19
62. Joung H-A, Ballard ZS, Wu J, Tseng DK, Teshome H, Zhang L, et al. Point-of-care serodiagnostic test for early-stage Lyme disease using a multiplexed paper-based immunoassay and machine learning. ACS Nano. (2020) 14:229–40. doi: 10.1021/acsnano.9b08151
63. Blauwkamp TA, Thair S, Rosen MJ, Blair L, Lindner MS, Vilfan ID, et al. Analytical and clinical validation of a microbial cell-free DNA sequencing test for infectious disease. Nat Microbiol. (2019) 4:663–74. doi: 10.1038/s41564-018-0349-6
64. Branda JA, Lemieux JE, Blair L, Ahmed AA, Hong DK, Bercovici S, et al. Detection of Borrelia burgdorferi cell-free DNA in human plasma samples for improved diagnosis of early Lyme borreliosis. Clin Infect Dis . (2020) ciaa858. doi: 10.1093/cid/ciaa858
65. Mosel MR, Carolan HE, Rebman AW, Castro S, Massire C, Ecker DJ, et al. Molecular testing of serial blood specimens from patients with early lyme disease during treatment reveals changing coinfection with mixtures of Borrelia burgdorferi genotypes. Antimicrob Agents Chemother. (2019) 63:e00237–19. doi: 10.1128/AAC.00237-19
66. Magni R, Espina BH, Shah K, Lepene B, Mayuga C, Douglas TA, et al. Application of Nanotrap technology for high sensitivity measurement of urinary outer surface protein A carboxyl-terminus domain in early stage Lyme borreliosis. J Transl Med. (2015) 13:346. doi: 10.1186/s12967-015-0701-z
67. Bouquet J, Soloski MJ, Swei A, Cheadle C, Federman S, Billaud J-N, et al. Longitudinal transcriptome analysis reveals a sustained differential gene expression signature in patients treated for acute Lyme disease. MBio. (2016) 7:e00100–16. doi: 10.1128/mBio.00100-16
68. Ma'ayan A, Clarke D, Rebman A, Bailey A, Wojciechowicz M, Jenkins S, et al. Predicting lyme disease from patients' peripheral blood mononuclear cells profiled with RNA-sequencing. Front Immunol. (2021) 12:452. doi: 10.3389/fimmu.2021.636289
69. Zhou Y, Qin S, Sun M, Tang L, Yan X, Kim T-K, et al. Measurement of organ-specific and acute-phase blood protein levels in early Lyme disease. J Proteome Res. (2020) 19:346–59. doi: 10.1021/acs.jproteome.9b00569
70. Reifert J, Kamath K, Bozekowski J, Lis E, Horn EJ, Granger D, et al. Serum epitope repertoire analysis enables early detection of Lyme disease with improved sensitivity in an expandable multiplex format. J Clin Microbiol . (2020) 59:e01836–20. doi: 10.1128/JCM.01836-20
71. Emerson RO, DeWitt WS, Vignali M, Gravley J, Hu JK, Osborne EJ, et al. Immunosequencing identifies signatures of cytomegalovirus exposure history and HLA-mediated effects on the T cell repertoire. Nat Genet. (2017) 49:659–65. doi: 10.1038/ng.3822
72. Molins CR, Ashton LV, Wormser GP, Hess AM, Delorey MJ, Mahapatra S, et al. Development of a metabolic biosignature for detection of early Lyme disease. Clin Infect Dis. (2015) 60:1767–75. doi: 10.1093/cid/civ185
73. Li K, Naviaux JC, Bright AT, Wang L, Naviaux RK. A robust, single-injection method for targeted, broad-spectrum plasma metabolomics. Metabolomics. (2017) 13:122. doi: 10.1007/s11306-017-1264-1
74. Pegalajar-Jurado A, Fitzgerald BL, Islam MN, Belisle JT, Wormser GP, Waller KS, et al. Identification of urine metabolites as biomarkers of early Lyme disease. Sci Rep. (2018) 8:12204. doi: 10.1038/s41598-018-29713-y
75. Fitzgerald BL, Molins CR, Islam MN, Graham B, Hove PR, Wormser GP, et al. Host metabolic response in early Lyme disease. J Proteome Res. (2020) 19:610–23. doi: 10.1021/acs.jproteome.9b00470
76. Callister SM, Jobe DA, Stuparic-Stancic A, Miyamasu M, Boyle J, Dattwyler RJ, et al. Detection of IFN-γ secretion by T cells collected before and after successful treatment of early Lyme disease. Clin Infect Dis. (2016) 62:1235–41. doi: 10.1093/cid/ciw112
77. Burlina PM, Joshi NJ, Ng E, Billings SD, Rebman AW, Aucott JN. Automated detection of erythema migrans and other confounding skin lesions via deep learning. Comput Biol Med. (2019) 105:151–6. doi: 10.1016/j.compbiomed.2018.12.007
78. Sell MG, Alcorta DA, Padilla AE, Nollner DW, Hasenkampf NR, Lambert HS, et al. Visualizing Borrelia burgdorferi infection using a small-molecule imaging probe. J Clin Microbiol. (2021) 59:e0231320. doi: 10.1128/JCM.02313-20
79. Asch ES, Bujak DI, Weiss M, Peterson MG, Weinstein A. Lyme disease: an infectious and postinfectious syndrome. J Rheumatol. (1994) 21:454–61.
PubMed Abstract | Google Scholar
80. Shadick NA, Phillips CB, Logigian EL, Steere AC, Kaplan RF, Berardi VP, et al. The long-term clinical outcomes of Lyme disease. A population-based retrospective cohort study. Ann Intern Med. (1994) 121:560–7. doi: 10.7326/0003-4819-121-8-199410150-00002
81. Moody KD, Adams RL, Barthold SW. Effectiveness of antimicrobial treatment against Borrelia burgdorferi infection in mice. Antimicrob Agents Chemother. (1994) 38:1567–72. doi: 10.1128/AAC.38.7.1567
82. Wormser GP, Nadelman RB, Dattwyler RJ, Dennis DT, Shapiro ED, Steere AC, et al. Practice guidelines for the treatment of Lyme disease. The Infectious Diseases Society of America. Clin Infect Dis. (2000) 31(Suppl. 1):1–14. doi: 10.1086/512462
CrossRef Full Text | Google Scholar
83. Lantos PM, Rumbaugh J, Bockenstedt LK, Falck-Ytter YT, Aguero-Rosenfeld ME, Auwaerter PG, et al. Clinical Practice Guidelines by the Infectious Diseases Society of America (IDSA), American Academy of Neurology (AAN), and American College of Rheumatology (ACR): 2020 Guidelines for the Prevention, Diagnosis and Treatment of Lyme Disease. Clin Infect Dis . (2020) 72:e1–e48. doi: 10.1093/cid/ciaa1215
84. Wu X, Sharma B, Niles S, O'Connor K, Schilling R, Matluck N, et al. Identifying vancomycin as an effective antibiotic for killing Borrelia burgdorferi . Antimicrob Agents Chemother . (2018) 62:e00237–19. doi: 10.1128/AAC.01201-18
85. Feng J, Wang T, Shi W, Zhang S, Sullivan D, Auwaerter PG, et al. Identification of novel activity against Borrelia burgdorferi persisters using an FDA approved drug library. Emerg Microbes Infect. (2014) 3:e49. doi: 10.1038/emi.2014.53
PubMed Abstract | CrossRef Full Text
86. Feng J, Auwaerter PG, Zhang Y. Drug combinations against Borrelia burgdorferi persisters in vitro : eradication achieved by using daptomycin, cefoperazone and doxycycline. PLoS ONE. (2015) 10:e0117207. doi: 10.1371/journal.pone.0117207
87. Feng J, Li T, Yee R, Yuan Y, Bai C, Cai M, et al. Stationary phase persister/biofilm microcolony of Borrelia burgdorferi causes more severe disease in a mouse model of Lyme arthritis: implications for understanding persistence, Post-treatment Lyme Disease Syndrome (PTLDS), and treatment failure. Discov Med. (2019) 27:125–38.
88. Feng J, Leone J, Schweig S, Zhang Y. Evaluation of natural and botanical medicines for activity against growing and non-growing forms of B. burgdorferi . Front Med . (2020) 7:6. doi: 10.3389/fmed.2020.00006
89. Zhang Y, Alvarez-Manzo H, Leone J, Schweig S, Zhang Y. Botanical medicines cryptolepis sanguinolenta, Artemisia annua, Scutellaria baicalensis, Polygonum cuspidatum , and Alchornea cordifolia demonstrate inhibitory activity against Babesia duncani . Front Cell Infect Microbiol . (2021) 11:624745. doi: 10.3389/fcimb.2021.624745
90. Feng J, Shi W, Miklossy J, Tauxe GM, McMeniman CJ, Zhang Y. Identification of essential oils with strong activity against stationary phase Borrelia burgdorferi . Antibiotics . (2018) 7:89. doi: 10.3390/antibiotics7040089
91. Feder HM, Johnson BJB, O'Connell S, Shapiro ED, Steere AC, Wormser GP, et al. A critical appraisal of “Chronic Lyme Disease.” N Engl J Med . (2007) 357:1422–30. doi: 10.1056/NEJMra072023
92. State of Connecticut. Circular Letter #12−32 . (1976). Available online at: https://portal.ct.gov/-/media/Departments-and-Agencies/DPH/dph/infectious_diseases/lyme/1976circularletterpdf.pdf?la=en (accessed July 22, 2021).
93. Barbour AG, Benach JL. Discovery of the Lyme disease agent. MBio. (2019) 10:e02166–19. doi: 10.1128/mBio.02166-19
94. Miller JB, Aucott JN. Stages of Lyme arthritis. J Clin Rheumatol . (2020). doi: 10.1097/RHU.0000000000001513. [Epub ahead of print].
95. Steere AC. Treatment of lyme arthritis. J Clin Rheumatol . (2019) 46:871–3. doi: 10.3899/jrheum.190320
96. Steere AC, Levin RE, Molloy PJ, Kalish RA, Abraham JH 3rd, Liu NY, et al. Treatment of Lyme arthritis. Arthritis Rheum. (1994) 37:878–88. doi: 10.1002/art.1780370616
97. Steere AC, Angelis SM. Therapy for Lyme arthritis: strategies for the treatment of antibiotic-refractory arthritis. Arthritis Rheum. (2006) 54:3079–86. doi: 10.1002/art.22131
98. Arvikar SL, Steere AC. Diagnosis and treatment of Lyme arthritis. Infect Dis Clin North Am. (2015) 29:269–80. doi: 10.1016/j.idc.2015.02.004
99. Horton DB, Taxter AJ, Davidow AL, Groh BP, Sherry DD, Rose CD. Intraarticular glucocorticoid injection as second-line treatment for Lyme arthritis in children. J Rheumatol. (2019) 46:952–9. doi: 10.3899/jrheum.180829
100. Stanek G, Wormser GP, Gray J, Strle F. Lyme borreliosis. Lancet. (2012) 379:461–73. doi: 10.1016/S0140-6736(11)60103-7
101. Lochhead RB, Strle K, Kim ND, Kohler MJ, Arvikar SL, Aversa JM, et al. MicroRNA expression shows inflammatory dysregulation and tumor-like proliferative responses in joints of patients with postinfectious Lyme arthritis. Arthritis Rheumatol. (2017) 69:1100–10. doi: 10.1002/art.40039
102. Jutras BL, Lochhead RB, Kloos ZA, Biboy J, Strle K, Booth CJ, et al. Borrelia burgdorferi peptidoglycan is a persistent antigen in patients with Lyme arthritis. Proc Natl Acad Sci USA. (2019) 116:13498–507. doi: 10.1073/pnas.1904170116
103. Bockenstedt LK, Gonzalez DG, Haberman AM, Belperron AA. Spirochete antigens persist near cartilage after murine Lyme borreliosis therapy. J Clin Invest. (2012) 122:2652–60. doi: 10.1172/JCI58813
104. Chandra AM, Keilp JG, Fallon BA. Correlates of perceived health-related quality of life in post-treatment Lyme encephalopathy. Psychosomatics. (2013) 54:552–9. doi: 10.1016/j.psym.2013.04.003
105. Johnson L, Wilcox S, Mankoff J, Stricker RB. Severity of chronic Lyme disease compared to other chronic conditions: a quality of life survey. PeerJ. (2014) 2:e322. doi: 10.7717/peerj.322
106. Marques A. Chronic Lyme disease: a review. Infect Dis Clin North Am. (2008) 22:341–60, vii–viii. doi: 10.1016/j.idc.2007.12.011
107. Aucott JN, Crowder LA, Kortte KB. Development of a foundation for a case definition of post-treatment Lyme disease syndrome. Int J Infect Dis. (2013) 17:e443–9. doi: 10.1016/j.ijid.2013.01.008
108. Aucott JN. Posttreatment Lyme disease syndrome. Infect Dis Clin North Am. (2015) 29:309–23. doi: 10.1016/j.idc.2015.02.012
109. DeLong A, Hsu M, Kotsoris H. Estimation of cumulative number of post-treatment Lyme disease cases in the US, 2016 and 2020. BMC Public Health. (2019) 19:352. doi: 10.1186/s12889-019-6681-9
110. Steere AC, Hutchinson GJ, Rahn DW, Sigal LH, Craft JE, DeSanna ET, et al. Treatment of the early manifestations of Lyme disease. Ann Intern Med. (1983) 99:22–6. doi: 10.7326/0003-4819-99-1-22
111. Dattwyler RJ, Halperin JJ. Failure of tetracycline therapy in early Lyme disease. Arthritis Rheum. (1987) 30:448–50. doi: 10.1002/art.1780300414
112. Donta ST. Tetracycline therapy for chronic Lyme disease. Clin Infect Dis. (1997) 25(Suppl. 1):S52–6. doi: 10.1086/516171
113. Shadick NA, Phillips CB, Sangha O, Logigian EL, Kaplan RF, Wright EA, et al. Musculoskeletal and neurologic outcomes in patients with previously treated Lyme disease. Ann Intern Med. (1999) 131:919–26. doi: 10.7326/0003-4819-131-12-199912210-00003
114. Kaplan RF, Trevino RP, Johnson GM, Levy L, Dornbush R, Hu LT, et al. Cognitive function in post-treatment Lyme disease: do additional antibiotics help? Neurology. (2003) 60:1916–22. doi: 10.1212/01.WNL.0000068030.26992.25
115. Bechtold KT, Rebman AW, Crowder LA, Johnson-Greene D, Aucott JN. Standardized symptom measurement of individuals with early Lyme disease over time. Arch Clin Neuropsychol. (2017) 32:129–41. doi: 10.1093/arclin/acw098
116. Horowitz RI, Freeman PR. Precision medicine: the role of the MSIDS model in defining, diagnosing, and treating chronic Lyme disease/Post Treatment Lyme Disease Syndrome and Other Chronic Illness: Part 2. Healthcare. (2018) 6:129. doi: 10.3390/healthcare6040129
117. Shor S, Green C, Szantyr B, Phillips S, Liegner K, Burrascano JJ Jr, et al. Chronic Lyme disease: an evidence-based definition by the ILADS Working Group. Antibiotics. (2019) 8:269. doi: 10.3390/antibiotics8040269
118. Rebman AW, Yang T, Aucott JN. Symptom heterogeneity and patient subgroup classification among US patients with post-treatment Lyme disease: an observational study. BMJ Open. (2021) 11:e040399. doi: 10.1136/bmjopen-2020-040399
119. Aucott JN, Soloski MJ, Rebman AW, Crowder LA, Lahey LJ, Wagner CA, et al. CCL19 as a chemokine risk factor for posttreatment Lyme disease syndrome: a prospective clinical cohort study. Clin Vaccine Immunol. (2016) 23:757–66. doi: 10.1128/CVI.00071-16
120. Strle K, Stupica D, Drouin EE, Steere AC, Strle F. Elevated levels of IL-23 in a subset of patients with post-lyme disease symptoms following erythema migrans. Clin Infect Dis. (2014) 58:372–80. doi: 10.1093/cid/cit735
121. Blum LK, Adamska JZ, Martin DS, Rebman AW, Elliott SE, Cao RRL, et al. Robust B cell responses predict rapid resolution of Lyme disease. Front Immunol. (2018) 9:1634. doi: 10.3389/fimmu.2018.01634
122. Stanek G, Reiter M. The expanding Lyme Borrelia complex–clinical significance of genomic species? Clin Microbiol Infect. (2011) 17:487–93. doi: 10.1111/j.1469-0691.2011.03492.x
123. Margos G, Fingerle V, Reynolds S. Borrelia bavariensis: vector switch, niche invasion, and geographical spread of a tick-borne bacterial parasite. Front Ecol Evol. (2019) 7:401. doi: 10.3389/fevo.2019.00401
124. Fingerle V, Schulte-Spechtel UC, Ruzic-Sabljic E, Leonhard S, Hofmann H, Weber K, et al. Epidemiological aspects and molecular characterization of Borrelia burgdorferi s.l. from southern Germany with special respect to the new species Borrelia spielmanii sp. nov. Int J Med Microbiol. (2008) 298:279–90. doi: 10.1016/j.ijmm.2007.05.002
125. Rudenko N, Golovchenko M, Ruzek D, Piskunova N, Mallátová N, Grubhoffer L. Molecular detection of Borrelia bissettii DNA in serum samples from patients in the Czech Republic with suspected borreliosis. FEMS Microbiol Lett. (2009) 292:274–81. doi: 10.1111/j.1574-6968.2009.01498.x
126. Girard YA, Fedorova N, Lane RS. Genetic diversity of Borrelia burgdorferi and detection of B. bissettii -like DNA in serum of north-coastal California residents. J Clin Microbiol. (2011) 49:945–54. doi: 10.1128/JCM.01689-10
127. Rudenko N, Golovchenko M, Vancova M, Clark K, Grubhoffer L, Oliver JH Jr. Isolation of live Borrelia burgdorferi sensu lato spirochaetes from patients with undefined disorders and symptoms not typical for Lyme borreliosis. Clin Microbiol Infect . (2016) 22:267.e9–15. doi: 10.1016/j.cmi.2015.11.009
128. Collares-Pereira M, Couceiro S, Franca I, Kurtenbach K, Schäfer SM, Vitorino L, et al. First isolation of Borrelia lusitaniae from a human patient. J Clin Microbiol. (2004) 42:1316–8. doi: 10.1128/JCM.42.3.1316-1318.2004
129. de Carvalho IL, Fonseca JE, Marques JG, Ullmann A, Hojgaard A, Zeidner N, et al. Vasculitis-like syndrome associated with Borrelia lusitaniae infection. Clin Rheumatol. (2008) 27:1587–91. doi: 10.1007/s10067-008-1012-z
130. Diza E, Papa A, Vezyri E, Tsounis S, Milonas I, Antoniadis A. Borrelia valaisiana in cerebrospinal fluid. Emerg Infect Dis. (2004) 10:1692–3. doi: 10.3201/eid1009.030439
131. Margos G, Sing A, Fingerle V. Published data do not support the notion that Borrelia valaisiana is human pathogenic. Infection. (2017) 45:567–9. doi: 10.1007/s15010-017-1032-1
132. Adeolu M, Gupta RS. A phylogenomic and molecular marker based proposal for the division of the genus Borrelia into two genera: the emended genus Borrelia containing only the members of the relapsing fever Borrelia, and the genus Borreliella gen. nov. containing the members of the Lyme disease Borrelia (Borrelia burgdorferi sensu lato complex). Antonie Van Leeuwenhoek. (2014) 105:1049–72. doi: 10.1007/s10482-014-0164-x
133. Barbour Ag. Borreliaceae. 1st ed. In: Whitman WB, Rainey F, Kämpfer P, Trujillo M, Chun J, DeVos P, et al., editors. Bergey's Manual of Systematics of Archaea and Bacteria . Hoboken, NJ: Wiley (2018). p. 1–22.
134. Cutler SJ. Relapsing fever borreliae: a global review. Clin Lab Med. (2015) 35:847–65. doi: 10.1016/j.cll.2015.07.001
135. Barbour AG, Adeolu M, Gupta RS. Division of the genus Borrelia into two genera (corresponding to Lyme disease and relapsing fever groups) reflects their genetic and phenotypic distinctiveness and will lead to a better understanding of these two groups of microbes (Margos et al.). There is inadequate evidence to support the division of the genus Borrelia. Int J Syst Evol Microbiol . (2017) 67:2058–67. doi: 10.1099/ijsem.0.001815
136. Margos G, Marosevic D, Cutler S, Derdakova M, Diuk-Wasser M, Emler S, et al. There is inadequate evidence to support the division of the genus Borrelia. Int J Syst Evol Microbiol . (2017) 67:1081−4. doi: 10.1099/ijsem.0.001717
137. Kingry LC, Anacker M, Pritt B, Bjork J, Respicio-Kingry L, Liu G, et al. Surveillance for and discovery of Borrelia Species in US Patients Suspected of Tickborne Illness. Clin Infect Dis. (2018) 66:1864–71. doi: 10.1093/cid/cix1107
138. Schotthoefer AM, Frost HM. Ecology and epidemiology of Lyme Borreliosis. Clin Lab Med. (2015) 35:723–43. doi: 10.1016/j.cll.2015.08.003
139. Rudenko N, Golovchenko M, Grubhoffer L, Oliver JH Jr. Updates on Borrelia burgdorferi sensu lato complex with respect to public health. Ticks Tick Borne Dis . (2011) 2:123–8. doi: 10.1016/j.ttbdis.2011.04.002
140. Wagemakers A, Staarink PJ, Sprong H, Hovius JWR. Borrelia miyamotoi : a widespread tick-borne relapsing fever spirochete. Trends Parasitol. (2015) 31:260–9. doi: 10.1016/j.pt.2015.03.008
141. Pritt BS, Mead PS, Johnson DKH, Neitzel DF, Respicio-Kingry LB, Davis JP, et al. Identification of a novel pathogenic Borrelia species causing Lyme borreliosis with unusually high spirochaetaemia: a descriptive study. Lancet Infect Dis. (2016) 16:556–64. doi: 10.1016/S1473-3099(15)00464-8
142. Kingry LC, Batra D, Replogle A, Rowe LA, Pritt BS, Petersen JM. Whole genome sequence and comparative genomics of the novel Lyme Borreliosis causing pathogen, Borrelia mayonii . PLoS ONE . (2016) 11:e0168994. doi: 10.1371/journal.pone.0168994
143. Schwartz I, Margos G, Casjens SR, Qiu W-G, Eggers CH. Multipartite genome of lyme disease borrelia: structure, variation and prophages. Curr Issues Mol Biol. (2020) 42:409–54. doi: 10.21775/cimb.042.409
144. Fraser CM, Casjens S, Huang WM, Sutton GG, Clayton R, Lathigra R, et al. Genomic sequence of a Lyme disease spirochaete, Borrelia burgdorferi . Nature . (1997) 390:580–6. doi: 10.1038/37551
145. Casjens S, Palmer N, van Vugt R, Huang WM, Stevenson B, Rosa P, et al. A bacterial genome in flux: the twelve linear and nine circular extrachromosomal DNAs in an infectious isolate of the Lyme disease spirochete Borrelia burgdorferi. Mol Microbiol. (2000) 35:490–516. doi: 10.1046/j.1365-2958.2000.01698.x
146. Schutzer SE, Fraser-Liggett CM, Casjens SR, Qiu W-G, Dunn JJ, Mongodin EF, et al. Whole-genome sequences of thirteen isolates of Borrelia burgdorferi . J Bacteriol . (2011) 193:1018–20. doi: 10.1128/JB.01158-10
147. Jabbari N, Glusman G, Joesch-Cohen LM, Reddy PJ, Moritz RL, Hood L, et al. Whole genome sequence and comparative analysis of Borrelia burgdorferi MM1. PLoS ONE. (2018) 13:e0198135. doi: 10.1371/journal.pone.0198135
148. Tyler S, Tyson S, Dibernardo A, Drebot M, Feil EJ, Graham M, et al. Whole genome sequencing and phylogenetic analysis of strains of the agent of Lyme disease Borrelia burgdorferi from Canadian emergence zones. Sci Rep. (2018) 8:10552. doi: 10.1038/s41598-018-28908-7
149. Di L, Pagan PE, Packer D, Martin CL, Akther S, Ramrattan G, et al. BorreliaBase: a phylogeny-centered browser of Borrelia genomes. BMC Bioinformatics. (2014) 15:233. doi: 10.1186/1471-2105-15-233
150. Di L, Wan Z, Akther S, Ying C, Larracuente A, Li L, et al. Genotyping and quantifying lyme pathogen strains by deep sequencing of the outer surface protein C (ospC) locus. J Clin Microbiol. (2018) 56:e00940–18. doi: 10.1128/JCM.00940-18
151. Casjens SR, Di L, Akther S, Mongodin EF, Luft BJ, Schutzer SE, et al. Primordial origin and diversification of plasmids in Lyme disease agent bacteria. BMC Genomics. (2018) 19:218. doi: 10.1186/s12864-018-4597-x
152. Margos G, Hepner S, Mang C, Marosevic D, Reynolds SE, Krebs S, et al. Lost in plasmids: next generation sequencing and the complex genome of the tick-borne pathogen Borrelia burgdorferi. BMC Genomics. (2017) 18:422. doi: 10.1186/s12864-017-3804-5
153. Mongodin EF, Casjens SR, Bruno JF, Xu Y, Drabek EF, Riley DR, et al. Inter- and intra-specific pan-genomes of Borrelia burgdorferi sensu lato: genome stability and adaptive radiation. BMC Genomics. (2013) 14:693. doi: 10.1186/1471-2164-14-693
154. Hanincova K, Mukherjee P, Ogden NH, Margos G, Wormser GP, Reed KD, et al. Multilocus sequence typing of Borrelia burgdorferi suggests existence of lineages with differential pathogenic properties in humans. PLoS ONE. (2013) 8:e73066. doi: 10.1371/journal.pone.0073066
155. Wang G, Liveris D, Mukherjee P, Jungnick S, Margos G, Schwartz I. Molecular typing of Borrelia burgdorferi . Curr Protoc Microbiol . (2014) 34:12C.5.1–31. doi: 10.1002/9780471729259.mc12c05s34
156. Cerar T, Strle F, Stupica D, Ruzic-Sabljic E, McHugh G, Steere AC, et al. Differences in genotype, clinical features, and inflammatory potential of Borrelia burgdorferi sensu stricto Strains from Europe and the United States. Emerg Infect Dis. (2016) 22:818–27. doi: 10.3201/eid2205.151806
157. Lin Y-P, Benoit V, Yang X, Martínez-Herranz R, Pal U, Leong JM. Strain-specific variation of the decorin-binding adhesin DbpA influences the tissue tropism of the lyme disease spirochete. PLoS Pathog. (2014) 10:e1004238. doi: 10.1371/journal.ppat.1004238
158. Troy EB, Lin T, Gao L, Lazinski DW, Lundt M, Camilli A, et al. Global Tn-seq analysis of carbohydrate utilization and vertebrate infectivity of Borrelia burgdorferi . Mol Microbiol . (2016) 101:1003–23. doi: 10.1111/mmi.13437
159. Ramsey ME, Hyde JA, Medina-Perez DN, Lin T, Gao L, Lundt ME, et al. A high-throughput genetic screen identifies previously uncharacterized Borrelia burgdorferi genes important for resistance against reactive oxygen and nitrogen species. PLoS Pathog. (2017) 13:e1006225. doi: 10.1371/journal.ppat.1006225
160. Phelan JP, Kern A, Ramsey ME, Lundt ME, Sharma B, Lin T, et al. Genome-wide screen identifies novel genes required for Borrelia burgdorferi survival in its Ixodes tick vector. PLoS Pathog. (2019) 15:e1007644. doi: 10.1371/journal.ppat.1007644
161. Barbour AG, Jasinskas A, Kayala MA, Davies DH, Steere AC, Baldi P, et al. A genome-wide proteome array reveals a limited set of immunogens in natural infections of humans and white-footed mice with Borrelia burgdorferi . Infect Immun . (2008) 76:3374–89. doi: 10.1128/IAI.00048-08
162. Dowdell AS, Murphy MD, Azodi C, Swanson SK, Florens L, Chen S, et al. Comprehensive spatial analysis of the Borrelia burgdorferi lipoproteome reveals a compartmentalization bias toward the bacterial surface. J Bacteriol. (2017) 199:e00658–16. doi: 10.1128/JB.00658-16
163. Setubal JC, Reis M, Matsunaga J, Haake DA. Lipoprotein computational prediction in spirochaetal genomes. Microbiology. (2006) 152:113–21. doi: 10.1099/mic.0.28317-0
164. Burgdorfer W, Barbour AG, Hayes SF, Benach JL, Grunwaldt E, Davis JP. Lyme disease-a tick-borne spirochetosis? Science. (1982) 216:1317–9. doi: 10.1126/science.7043737
165. Talagrand-Reboul E, Westermann B, Raess MA, Schnell G, Cantero P, Barthel C, et al. Proteomic as an exploratory approach to develop vaccines against tick-borne diseases using Lyme borreliosis as a test case. Vaccines. (2020) 8:463. doi: 10.3390/vaccines8030463
166. Angel TE, Luft BJ, Yang X, Nicora CD, Camp DG 2nd, Jacobs JM, et al. Proteome analysis of Borrelia burgdorferi response to environmental change. PLoS ONE. (2010) 5:e13800. doi: 10.1371/journal.pone.0013800
167. Schnell G, Boeuf A, Jaulhac B, Boulanger N, Collin E, Barthel C, et al. Proteomic analysis of three Borrelia burgdorferi sensu lato native species and disseminating clones: relevance for Lyme vaccine design. Proteomics. (2015) 15:1280–290. doi: 10.1002/pmic.201400177
168. Deutsch EW, Mendoza L, Shteynberg D, Slagel J, Sun Z, Moritz RL. Trans-proteomic pipeline, a standardized data processing pipeline for large-scale reproducible proteomics informatics. Proteomics Clin Appl. (2015) 9:745–54. doi: 10.1002/prca.201400164
169. Tokarz R, Tagliafierro T, Sameroff S, Cucura DM, Oleynik A, Che X, et al. Microbiome analysis of Ixodes scapularis ticks from New York and Connecticut. Ticks Tick Borne Dis . (2019) 10:894–900. doi: 10.1016/j.ttbdis.2019.04.011
170. Crowder CD, Matthews HE, Schutzer S, Rounds MA, Luft BJ, Nolte O, et al. Genotypic variation and mixtures of Lyme Borrelia in Ixodes ticks from North America and Europe. PLoS ONE. (2010) 5:e10650. doi: 10.1371/journal.pone.0010650
171. Guttman DS, Wang PW, Wang IN, Bosler EM, Luft BJ, Dykhuizen DE. Multiple infections of Ixodes scapularis ticks by Borrelia burgdorferi as revealed by single-strand conformation polymorphism analysis. J Clin Microbiol. (1996) 34:652–6. doi: 10.1128/jcm.34.3.652-656.1996
172. Seinost G, Golde WT, Berger BW, Dunn JJ, Qiu D, Dunkin DS, et al. Infection with multiple strains of Borrelia burgdorferi sensu stricto in patients with Lyme disease. Arch Dermatol. (1999) 135:1329–33. doi: 10.1001/archderm.135.11.1329
173. Liveris D, Varde S, Iyer R, Koenig S, Bittker S, Cooper D, et al. Genetic diversity of Borrelia burgdorferi in lyme disease patients as determined by culture versus direct PCR with clinical specimens. J Clin Microbiol. (1999) 37:565–9. doi: 10.1128/JCM.37.3.565-569.1999
174. Keesing F, Brunner J, Duerr S, Killilea M, Logiudice K, Schmidt K, et al. Hosts as ecological traps for the vector of Lyme disease. Proc Biol Sci. (2009) 276:3911–9. doi: 10.1098/rspb.2009.1159
175. Stewart PE, Rosa PA. Physiologic and genetic factors influencing the zoonotic cycle of Borrelia burgdorferi . In: Adler B, editor. Spirochete Biology: The Post Genomic Era . Cham: Springer International Publishing (2018). p. 63–82.
176. Scoles GA, Papero M, Beati L, Fish D. A relapsing fever group spirochete transmitted by Ixodes scapularis ticks. Vector Borne Zoonotic Dis. (2001) 1:21–34. doi: 10.1089/153036601750137624
177. Rollend L, Fish D, Childs JE. Transovarial transmission of Borrelia spirochetes by Ixodes scapularis: a summary of the literature and recent observations. Ticks Tick Borne Dis. (2013) 4:46–51. doi: 10.1016/j.ttbdis.2012.06.008
178. Kurokawa C, Lynn GE, Pedra JHF, Pal U, Narasimhan S, Fikrig E. Interactions between Borrelia burgdorferi and ticks. Nat Rev Microbiol . (2020) 18:587–600. doi: 10.1038/s41579-020-0400-5
179. Ross BD, Hayes B, Radey MC, Lee X, Josek T, Bjork J, et al. Ixodes scapularis does not harbor a stable midgut microbiome. ISME J. (2018) 12:2596–607. doi: 10.1038/s41396-018-0161-6
180. Stewart PE, Bloom ME. Sharing the ride: ixodes scapularis symbionts and their interactions. Front Cell Infect Microbiol. (2020) 10:142. doi: 10.3389/fcimb.2020.00142
181. Narasimhan S, Rajeevan N, Liu L, Zhao YO, Heisig J, Pan J, et al. Gut microbiota of the tick vector Ixodes scapularis modulate colonization of the Lyme disease spirochete. Cell Host Microbe. (2014) 15:58–71. doi: 10.1016/j.chom.2013.12.001
182. Landesman WJ, Mulder K, Fredericks LP, Allan BF. Cross-kingdom analysis of nymphal-stage Ixodes scapularis microbial communities in relation to Borrelia burgdorferi infection and load. FEMS Microbiol Ecol. (2019) 95:fiz167. doi: 10.1093/femsec/fiz167
183. Nuttall PA. Wonders of tick saliva. Ticks Tick Borne Dis. (2019) 10:470–81. doi: 10.1016/j.ttbdis.2018.11.005
184. Šimo L, Kazimirova M, Richardson J, Bonnet SI. The essential role of tick salivary glands and saliva in tick feeding and pathogen transmission. Front Cell Infect Microbiol. (2017) 7:281. doi: 10.3389/fcimb.2017.00281
185. Wikel S. Ticks and tick-borne pathogens at the cutaneous interface: host defenses, tick countermeasures, and a suitable environment for pathogen establishment. Front Microbiol. (2013) 4:337. doi: 10.3389/fmicb.2013.00337
186. Vora A, Taank V, Dutta SM, Anderson JF, Fish D, Sonenshine DE, et al. Ticks elicit variable fibrinogenolytic activities upon feeding on hosts with different immune backgrounds. Sci Rep. (2017) 7:44593. doi: 10.1038/srep44593
187. Ribeiro JM, Mather TN. Ixodes scapularis: salivary kininase activity is a metallo dipeptidyl carboxypeptidase. Exp Parasitol. (1998) 89:213–21. doi: 10.1006/expr.1998.4296
188. Paesen GC, Adams PL, Nuttall PA, Stuart DL. Tick histamine-binding proteins: lipocalins with a second binding cavity. Biochim Biophys Acta. (2000) 1482:92–101. doi: 10.1016/S0167-4838(00)00168-0
189. Zhou W, Tahir F, Wang JC-Y, Woodson M, Sherman MB, Karim S, et al. Discovery of exosomes from tick saliva and salivary glands reveals therapeutic roles for CXCL12 and IL-8 in wound healing at the tick-human skin interface. Front Cell Dev Biol. (2020) 8:554. doi: 10.3389/fcell.2020.00554
190. Bernard Q, Gallo RL, Jaulhac B, Nakatsuji T, Luft B, Yang X, et al. Ixodes tick saliva suppresses the keratinocyte cytokine response to TLR2/TLR3 ligands during early exposure to Lyme borreliosis. Exp Dermatol. (2016) 25:26–31. doi: 10.1111/exd.12853
191. Scholl DC, Embers ME, Caskey JR, Kaushal D, Mather TN, Buck WR, et al. Immunomodulatory effects of tick saliva on dermal cells exposed to Borrelia burgdorferi , the agent of Lyme disease. Parasit Vect. (2016) 9:394. doi: 10.1186/s13071-016-1638-7
192. Kim TK, Tirloni L, Pinto AFM, Moresco J, Yates JR III, da Silva Vaz I Jr, et al. Ixodes scapularis tick saliva proteins sequentially secreted every 24 h during blood feeding. PLoS Negl Trop Dis. (2016) 10:e0004323. doi: 10.1371/journal.pntd.0004323
193. Takeuchi O, Akira S. Pattern recognition receptors and inflammation. Cell. (2010) 140:805–20. doi: 10.1016/j.cell.2010.01.022
194. Salazar JC, Pope CD, Sellati TJ, Feder HM Jr, Kiely TG, Dardick KR, et al. Coevolution of markers of innate and adaptive immunity in skin and peripheral blood of patients with erythema migrans. J Immunol. (2003) 171:2660–70. doi: 10.4049/jimmunol.171.5.2660
195. Petnicki-Ocwieja T, Kern A. Mechanisms of Borrelia burgdorferi internalization and intracellular innate immune signaling. Front Cell Infect Microbiol. (2014) 4:175. doi: 10.3389/fcimb.2014.00175
196. Gupta A, Arora G, Rosen CE, Kloos Z, Cao Y, Cerny J, et al. A human secretome library screen reveals a role for peptidoglycan recognition protein 1 in Lyme borreliosis. PLoS Pathog. (2020) 16:e1009030. doi: 10.1371/journal.ppat.1009030
197. Hawley KL, Olson CM Jr, Iglesias-Pedraz JM, Navasa N, Cervantes JL, Caimano MJ, et al. CD14 cooperates with complement receptor 3 to mediate MyD88-independent phagocytosis of Borrelia burgdorferi . Proc Natl Acad Sci USA . (2012) 109:1228–32. doi: 10.1073/pnas.1112078109
198. Hawley KL, Martín-Ruiz I, Iglesias-Pedraz JM, Berwin B, Anguita J. CD14 targets complement receptor 3 to lipid rafts during phagocytosis of Borrelia burgdorferi . Int J Biol Sci . (2013) 9:803–10. doi: 10.7150/ijbs.7136
199. Hawley KL, Olson CM Jr, Carreras-González A, Navasa N, Anguita J. Serum C3 enhances complement receptor 3-mediated phagocytosis of Borrelia burgdorferi . Int J Biol Sci . (2015) 11:1269–71. doi: 10.7150/ijbs.13395
200. Carreras-González A, Barriales D, Palacios A, Montesinos-Robledo M, Navasa N, Azkargorta M, et al. Regulation of macrophage activity by surface receptors contained within Borrelia burgdorferi -enriched phagosomal fractions. PLoS Pathog. (2019) 15:e1008163. doi: 10.1371/journal.ppat.1008163
201. Hovius JWR, Bijlsma MF, van der Windt GJW, Wiersinga WJ, Boukens BJD, Coumou J, et al. The urokinase receptor (uPAR) facilitates clearance of Borrelia burgdorferi . PLoS Pathog . (2009) 5:e1000447. doi: 10.1371/journal.ppat.1000447
202. Petnicki-Ocwieja T, Chung E, Acosta DI, Ramos LT, Shin OS, Ghosh S, et al. TRIF mediates Toll-like receptor 2-dependent inflammatory responses to Borrelia burgdorferi . Infect Immun . (2013) 81:402–10. doi: 10.1128/IAI.00890-12
203. Krupna-Gaylord MA, Liveris D, Love AC, Wormser GP, Schwartz I, Petzke MM. Induction of type I and type III interferons by Borrelia burgdorferi correlates with pathogenesis and requires linear plasmid 36. PLoS ONE. (2014) 9:e100174. doi: 10.1371/journal.pone.0100174
204. Cohen TS, Parker D. Microbial pathogenesis and type III interferons. Cytokine Growth Factor Rev. (2016) 29:45–51. doi: 10.1016/j.cytogfr.2016.02.005
205. Syedbasha M, Egli A. Interferon lambda: modulating immunity in infectious diseases. Front Immunol. (2017) 8:119. doi: 10.3389/fimmu.2017.00119
206. Cervantes JL, La Vake CJ, Weinerman B, Luu S, O'Connell C, Verardi PH, et al. Human TLR8 is activated upon recognition of Borrelia burgdorferi RNA in the phagosome of human monocytes. J Leukoc Biol. (2013) 94:1231–41. doi: 10.1189/jlb.0413206
207. Moore MW, Cruz AR, LaVake CJ, Marzo AL, Eggers CH, Salazar JC, et al. Phagocytosis of Borrelia burgdorferi and Treponema pallidum potentiates innate immune activation and induces gamma interferon production. Infect Immun. (2007) 75:2046–62. doi: 10.1128/IAI.01666-06
208. Love AC, Schwartz I, Petzke MM. Borrelia burgdorferi RNA induces type I and III interferons via Toll-like receptor 7 and contributes to production of NF-κB-dependent cytokines. Infect Immun. (2014) 82:2405–16. doi: 10.1128/IAI.01617-14
209. Soloski MJ, Crowder LA, Lahey LJ, Wagner CA, Robinson WH, Aucott JN. Serum inflammatory mediators as markers of human Lyme disease activity. PLoS ONE. (2014) 9:e93243. doi: 10.1371/journal.pone.0093243
210. Uhde M, Ajamian M, Li X, Wormser GP, Marques A, Alaedini A. Expression of C-reactive protein and serum amyloid A in early to late manifestations of Lyme disease. Clin Infect Dis. (2016) 63:1399–404. doi: 10.1093/cid/ciw599
211. McPherson RA. Specific proteins. In: McPherson RA, Pincus MR, editors. Henry's Clinical Diagnosis and Management by Laboratory Methods . St. Louis, MO: Elsevier Health Sciences (2017). p. 253–66.e2. Available online at: https://www.google.com/books/edition/Henry_s_Clinical_Diagnosis_and_Managemen/xAzhCwAAQBAJ?hl=en (accessed July 22, 2021).
212. Nehring SM, Goyal A, Bansal P, Patel BC. C Reactive Protein (CRP) . Treasure Island, FL: StatPearls Publishing (2020).
213. Kushner I, Rzewnicki D, Samols D. What does minor elevation of C-reactive protein signify? Am J Med. (2006) 119:166.e17–28. doi: 10.1016/j.amjmed.2005.06.057
214. Kushner I, Antonelli MJ. What should we regard as an “elevated” C-reactive protein level? Ann Intern Med . (2015) 163:326. doi: 10.7326/L15-5126
215. Greenland P, Alpert JS, Beller GA, Benjamin EJ, Budoff MJ, Fayad ZA, et al. 2010 ACCF/AHA guideline for assessment of cardiovascular risk in asymptomatic adults: a report of the American College of Cardiology Foundation/American Heart Association Task Force on Practice Guidelines. J Am Coll Cardiol . (2010) 56:e50–103. doi: 10.1016/j.jacc.2010.09.002
216. Lochhead RB, Arvikar SL, Aversa JM. Robust interferon signature and suppressed tissue repair gene expression in synovial tissue from patients with postinfectious, Borrelia burgdorferi -induced Lyme arthritis. Cell Microbiol . (2019) 21:e12954. doi: 10.1111/cmi.12954
217. Kuwabara T, Ishikawa F, Yasuda T, Aritomi K, Nakano H, Tanaka Y, et al. CCR7 ligands are required for development of experimental autoimmune encephalomyelitis through generating IL-23-dependent Th17 cells. J Immunol. (2009) 183:2513–21. doi: 10.4049/jimmunol.0800729
218. Root-Bernstein R, Fairweather D. Complexities in the relationship between infection and autoimmunity. Curr Allergy Asthma Rep. (2014) 14:407. doi: 10.1007/s11882-013-0407-3
219. Badloe FMS, Janmohamed SR, Ring J, Gutermuth J. Microbial triggers in autoimmunity, severe allergy, and autoallergy. In: Schmidt-Weber CB, editor. Allergy Prevention and Exacerbation . Cham: Springer International Publishing (2017). p. 11–26.
220. Steere AC, Dwyer E, Winchester R. Association of chronic Lyme arthritis with HLA-DR4 and HLA-DR2 alleles. N Engl J Med. (1990) 323:219–23. doi: 10.1056/NEJM199007263230402
221. Gross DM, Forsthuber T, Tary-Lehmann M, Etling C, Ito K, Nagy ZA, et al. Identification of LFA-1 as a candidate autoantigen in treatment-resistant Lyme arthritis. Science. (1998) 281:703–6. doi: 10.1126/science.281.5377.703
222. Steere AC, Klitz W, Drouin EE, Falk BA, Kwok WW, Nepom GT, et al. Antibiotic-refractory Lyme arthritis is associated with HLA-DR molecules that bind a Borrelia burgdorferi peptide. J Exp Med. (2006) 203:961–71. doi: 10.1084/jem.20052471
223. Ghosh S, Seward R, Costello CE, Stollar BD, Huber BT. Autoantibodies from synovial lesions in chronic, antibiotic treatment-resistant Lyme arthritis bind cytokeratin-10. J Immunol. (2006) 177:2486–94. doi: 10.4049/jimmunol.177.4.2486
224. Drouin EE, Glickstein L, Kwok WW, Nepom GT, Steere AC. Human homologues of a Borrelia T cell epitope associated with antibiotic-refractory Lyme arthritis. Mol Immunol. (2008) 45:180–9. doi: 10.1016/j.molimm.2007.04.017
225. Seward RJ, Drouin EE, Steere AC, Costello CE. Peptides presented by HLA-DR molecules in synovia of patients with rheumatoid arthritis or antibiotic-refractory Lyme arthritis. Mol Cell Proteomics. (2011) 10:M110.002477. doi: 10.1074/mcp.M110.002477
226. Londoño D, Cadavid D, Drouin EE, Strle K, McHugh G, Aversa JM, et al. Antibodies to endothelial cell growth factor and obliterative microvascular lesions in the synovium of patients with antibiotic-refractory lyme arthritis. Arthritis Rheumatol. (2014) 66:2124–33. doi: 10.1002/art.38618
227. Pianta A, Drouin EE, Crowley JT, Arvikar S, Strle K, Costello CE, et al. Annexin A2 is a target of autoimmune T and B cell responses associated with synovial fibroblast proliferation in patients with antibiotic-refractory Lyme arthritis. Clin Immunol. (2015) 160:336–41. doi: 10.1016/j.clim.2015.07.005
228. Sulka KB, Strle K, Crowley JT, Lochhead RB, Anthony R, Steere AC. Correlation of Lyme disease-associated IgG4 autoantibodies with synovial pathology in antibiotic-refractory Lyme arthritis. Arthritis Rheumatol. (2018) 70:1835–46. doi: 10.1002/art.40566
229. Crowley JT, Strle K, Drouin EE, Pianta A, Arvikar SL, Wang Q, et al. Matrix metalloproteinase-10 is a target of T and B cell responses that correlate with synovial pathology in patients with antibiotic-refractory Lyme arthritis. J Autoimmun. (2016) 69:24–37. doi: 10.1016/j.jaut.2016.02.005
230. Zuniga M, Gomes C, Carsons SE, Bender MT, Cotzia P, Miao QR, et al. Autoimmunity to the lung protective phospholipid-binding protein Annexin A2 predicts mortality among hospitalized COVID-19 patients. Eur Respir J . (2021) 2100918. doi: 10.1183/13993003.00918-2021
231. Dworkin J. The medium is the message: interspecies and interkingdom signaling by peptidoglycan and related bacterial glycans. Annu Rev Microbiol. (2014) 68:137–54. doi: 10.1146/annurev-micro-091213-112844
232. Wolf AJ, Underhill DM. Peptidoglycan recognition by the innate immune system. Nat Rev Immunol. (2018) 18:243–54. doi: 10.1038/nri.2017.136
233. Irazoki O, Hernandez SB, Cava F. Peptidoglycan muropeptides: release, perception, and functions as signaling molecules. Front Microbiol. (2019) 10:500. doi: 10.3389/fmicb.2019.00500
234. Chan JM, Dillard JP. Attention seeker: production, modification, and release of inflammatory peptidoglycan fragments in neisseria species. J Bacteriol. (2017) 199:e00354–17. doi: 10.1128/JB.00354-17
235. Wolf AJ, Reyes CN, Liang W, Becker C, Shimada K, Wheeler ML, et al. Hexokinase is an innate immune receptor for the detection of bacterial peptidoglycan. Cell. (2016) 166:624–36. doi: 10.1016/j.cell.2016.05.076
236. Boneca IG. The role of peptidoglycan in pathogenesis. Curr Opin Microbiol. (2005) 8:46–53. doi: 10.1016/j.mib.2004.12.008
237. Schrijver IA, Melief MJ, Markusse HM, Van Aelst I, Opdenakker G, Hazenberg MP, et al. Peptidoglycan from sterile human spleen induces T-cell proliferation and inflammatory mediators in rheumatoid arthritis patients and healthy subjects. Rheumatology. (2001) 40:438–46. doi: 10.1093/rheumatology/40.4.438
238. Schrijver IA, van Meurs M, Melief MJ, Wim Ang C, Buljevac D, Ravid R, et al. Bacterial peptidoglycan and immune reactivity in the central nervous system in multiple sclerosis. Brain. (2001) 124:1544–54. doi: 10.1093/brain/124.8.1544
239. Kriesel JD, Bhetariya P, Wang Z-M, Renner D, Palmer C, Fischer KF. Spectrum of microbial sequences and a bacterial cell wall antigen in primary demyelination brain specimens obtained from living patients. Sci Rep. (2019) 9:1387. doi: 10.1038/s41598-018-38198-8
240. Azuma I, Taniyama T, Yamamura Y, Yanagihara Y, Hattori Y. Chemical studies on the cell walls of Leptorspira biflexa strain Urawa and Treponema pallidum strain Reiter. Jpn J Microbiol. (1975) 19:45–51. doi: 10.1111/j.1348-0421.1975.tb00846.x
241. Yanagihara Y, Kamisango K, Yasuda S, Kobayashi S, Mifuchi I, Azuma I, et al. Chemical compositions of cell walls and polysaccharide fractions of spirochetes. Microbiol Immunol. (1984) 28:535–44. doi: 10.1111/j.1348-0421.1984.tb00706.x
242. Beck G, Benach JL, Habicht GS. Isolation, preliminary chemical characterization, and biological activity of Borrelia burgdorferi peptidoglycan. Biochem Biophys Res Commun. (1990) 167:89–95. doi: 10.1016/0006-291X(90)91734-A
243. Sharma B, Brown AV, Matluck NE, Hu LT, Lewis K. Borrelia burgdorferi, the causative agent of Lyme disease, forms drug-tolerant persister cells. Antimicrob Agents Chemother. (2015) 59:4616–24. doi: 10.1128/AAC.00864-15
244. Balaban NQ, Helaine S, Lewis K, Ackermann M, Aldridge B, Andersson DI, et al. Definitions and guidelines for research on antibiotic persistence. Nat Rev Microbiol . (2019) 17:441–8. doi: 10.1038/s41579-019-0207-4
245. Gollan B, Grabe G, Michaux C, Helaine S. Bacterial persisters and infection: past, present, and progressing. Annu Rev Microbiol. (2019) 73:359–85. doi: 10.1146/annurev-micro-020518-115650
246. Lewis K. (editor). Persister Cells and Infectious Disease (1st ed.). Switzerland: Springer (2019).
247. Lantos PM, Auwaerter PG, Wormser GP. A systematic review of Borrelia burgdorferi morphologic variants does not support a role in chronic Lyme disease. Clin Infect Dis. (2014) 58:663–71. doi: 10.1093/cid/cit810
248. Alvarez-Ortega C, Wiegand I, Olivares J, Hancock REW, Martínez JL. The intrinsic resistome of Pseudomonas aeruginosa to β-lactams. Virulence. (2011) 2:144–6. doi: 10.4161/viru.2.2.15014
249. Georghiou SB, Magana M, Garfein RS, Catanzaro DG, Catanzaro A, Rodwell TC. Evaluation of genetic mutations associated with Mycobacterium tuberculosis resistance to amikacin, kanamycin and capreomycin: a systematic review. PLoS ONE. (2012) 7:e33275. doi: 10.1371/journal.pone.0033275
250. Rattan A, Kalia A, Ahmad N. Multidrug-resistant Mycobacterium tuberculosis : molecular perspectives. Emerg Infect Dis. (1998) 4:195–209. doi: 10.3201/eid0402.980207
251. Bunikis I, Denker K, Ostberg Y, Andersen C, Benz R, Bergström S. An RND-type efflux system in Borrelia burgdorferi is involved in virulence and resistance to antimicrobial compounds. PLoS Pathog. (2008) 4:e1000009. doi: 10.1371/journal.ppat.1000009
252. Lewis K. Persister cells, dormancy and infectious disease. Nat Rev Microbiol. (2007) 5:48–56. doi: 10.1038/nrmicro1557
253. Lewis K. Persister cells. Annu Rev Microbiol. (2010) 64:357–72. doi: 10.1146/annurev.micro.112408.134306
254. Caskey JR, Embers ME. Persister development by Borrelia burgdorferi populations in vitro . Antimicrob Agents Chemother. (2015) 59:6288–95. doi: 10.1128/AAC.00883-15
255. Gengenbacher M, Kaufmann SHE. Mycobacterium tuberculosis: success through dormancy. FEMS Microbiol Rev. (2012) 36:514–32. doi: 10.1111/j.1574-6976.2012.00331.x
256. Singh AE, Romanowski B. Syphilis: review with emphasis on clinical, epidemiologic, and some biologic features. Clin Microbiol Rev. (1999) 12:187–209. doi: 10.1128/CMR.12.2.187
257. Cabello FC, Godfrey HP, Bugrysheva JV, Newman SA. Sleeper cells: the stringent response and persistence in the Borreliella (Borrelia) burgdorferi enzootic cycle. Environ Microbiol. (2017) 19:3846–62. doi: 10.1111/1462-2920.13897
258. Caskey JR, Hasenkampf NR, Martin DS, Chouljenko VN, Subramanian R, Cheslock MA, et al. The functional and molecular effects of doxycycline treatment on Borrelia burgdorferi phenotype. Front Microbiol. (2019) 10:690. doi: 10.3389/fmicb.2019.00690
259. Mayer L, Merz S. An appraisal of “chronic Lyme disease.” New Engl J Med . (2008)358:428–31. doi: 10.1056/NEJMc073063
260. Stricker RB. Counterpoint: long-term antibiotic therapy improves persistent symptoms associated with lyme disease. Clin Infect Dis. (2007) 45:149–57. doi: 10.1086/518853
261. Auwaerter PG. Point: antibiotic therapy is not the answer for patients with persisting symptoms attributable to lyme disease. Clin Infect Dis. (2007) 45:143–8. doi: 10.1086/518854
262. Aucott JN, Rebman AW, Crowder LA, Kortte KB. Post-treatment Lyme disease syndrome symptomatology and the impact on life functioning: is there something here? Qual Life Res. (2013) 22:75–84. doi: 10.1007/s11136-012-0126-6
263. Klempner MS. Controlled trials of antibiotic treatment in patients with post-treatment chronic Lyme disease. Vector Borne Zoonotic Dis. (2002) 2:255–63. doi: 10.1089/153036602321653842
264. Berende A, ter Hofstede HJM, Vos FJ, van Middendorp H, Vogelaar ML, Tromp M, et al. Randomized trial of longer-term therapy for symptoms attributed to Lyme disease. N Engl J Med. (2016) 374:1209–20. doi: 10.1056/NEJMoa1505425
265. Klempner MS, Hu LT, Evans J, Schmid CH, Johnson GM, Trevino RP, et al. Two controlled trials of antibiotic treatment in patients with persistent symptoms and a history of Lyme disease. N Engl J Med. (2001) 345:85–92. doi: 10.1056/NEJM200107123450202
266. Embers M, Gadila S, Rosoklija G, Dwork A, Fallon B. Detection of Borrelia spirochetes in autopsy specimens: validation and a case study. Front Neurol. (2021) 12:707. doi: 10.3389/fneur.2021.628045
267. Straubinger RK, Summers BA, Chang YF, Appel MJ. Persistence of Borrelia burgdorferi in experimentally infected dogs after antibiotic treatment. J Clin Microbiol. (1997) 35:111–6. doi: 10.1128/jcm.35.1.111-116.1997
268. Embers ME, Barthold SW, Borda JT, Bowers L, Doyle L, Hodzic E, et al. Persistence of Borrelia burgdorferi in rhesus macaques following antibiotic treatment of disseminated infection. PLoS ONE. (2012) 7:e29914. doi: 10.1371/annotation/4cafed66-fb84-4589-a001-131d9c50aea6
269. Hodzic E, Imai D, Feng S, Barthold SW. Resurgence of persisting non-cultivable Borrelia burgdorferi following antibiotic treatment in mice. PLoS ONE. (2014) 9:e86907. doi: 10.1371/journal.pone.0086907
270. Marques A, Telford SR, Turk S-P, Chung E, Williams C, Dardick K, et al. Xenodiagnosis to detect Borrelia burgdorferi infection: a first-in-human study. Clin Infect Dis. (2014) 58:937–45. doi: 10.1093/cid/cit939
271. Bockenstedt LK, Radolf JD. Xenodiagnosis for posttreatment Lyme disease syndrome: resolving the conundrum or adding to it? Clin Infect Dis . (2014) 58:946–8. doi: 10.1093/cid/cit942
272. Bockenstedt LK, Mao J, Hodzic E, Barthold SW, Fish D. Detection of attenuated, noninfectious spirochetes in Borrelia burgdorferi -infected mice after antibiotic treatment. J Infect Dis. (2002) 186:1430–7. doi: 10.1086/345284
273. Hodzic E, Feng S, Holden K, Freet KJ, Barthold SW. Persistence of Borrelia burgdorferi following antibiotic treatment in mice. Antimicrob Agents Chemother. (2008) 52:1728–36. doi: 10.1128/AAC.01050-07
274. Barthold SW, Hodzic E, Imai DM, Feng S, Yang X, Luft BJ. Ineffectiveness of tigecycline against persistent Borrelia burgdorferi . Antimicrob Agents Chemother . (2010) 54:643–51. doi: 10.1128/AAC.00788-09
275. Embers ME, Hasenkampf NR, Jacobs MB, Tardo AC, Doyle-Meyers LA, Philipp MT, et al. Variable manifestations, diverse seroreactivity and post-treatment persistence in non-human primates exposed to Borrelia burgdorferi by tick feeding. PLoS ONE. (2017) 12:e0189071. doi: 10.1371/journal.pone.0189071
276. Crossland NA, Alvarez X, Embers ME. Late disseminated Lyme disease: associated pathology and spirochete persistence posttreatment in rhesus macaques. Am J Pathol. (2018) 188:672–82. doi: 10.1016/j.ajpath.2017.11.005
277. Barbour A. Remains of infection. J Clin Investig . (2012) 122:2344−6. doi: 10.1172/JCI63975
278. Wormser GP, Baker PJ, O'Connell S, Pachner AR, Schwartz I, Shapiro ED. Critical analysis of treatment trials of rhesus macaques infected with Borrelia burgdorferi reveals important flaws in experimental design. Vector Borne Zoon Dis. (2012) 12:535–8. doi: 10.1089/vbz.2012.1012
279. Ayrapetyan M, Williams T, Oliver JD. Relationship between the viable but nonculturable state and antibiotic persister cells. J Bacteriol. (2018) 200:e00249–18. doi: 10.1128/JB.00249-18
280. Ramamurthy T, Ghosh A, Pazhani GP, Shinoda S. Current perspectives on viable but non-culturable (VBNC) pathogenic bacteria. Front Public Health. (2014) 2:103. doi: 10.3389/fpubh.2014.00103
281. Li L, Mendis N, Trigui H, Oliver JD, Faucher SP. The importance of the viable but non-culturable state in human bacterial pathogens. Front Microbiol. (2014) 5:258. doi: 10.3389/fmicb.2014.00258
282. Rice SA, McDougald D, Kjelleberg S. Vibrio vulnificus: a physiological and genetic approach to the viable but nonculturable response. J Infect Chemother. (2000) 6:115–20. doi: 10.1007/PL00012150
283. Orruño M, Kaberdin VR, Arana I. Survival strategies of Escherichia coli and Vibrio spp.: contribution of the viable but nonculturable phenotype to their stress-resistance and persistence in adverse environments. World J Microbiol Biotechnol. (2017) 33:45. doi: 10.1007/s11274-017-2218-5
284. Fischhoff IR, Keesing F, Ostfeld RS. Risk factors for bites and diseases associated with black-legged ticks: a meta-analysis. Am J Epidemiol. (2019) 188:1742–50. doi: 10.1093/aje/kwz130
285. Preventing tick bites. In: CDC . (2019). Available online at: https://www.cdc.gov/ticks/avoid/on_people.html (accessed June 12, 2019).
286. Beard CB. Lyme disease prevention and control - the way forward. Can Commun Dis Rep. (2014) 40:91–4. doi: 10.14745/ccdr.v40i05a04
287. Ostfeld RS, Levi T, Keesing F, Oggenfuss K, Canham CD. Tick-borne disease risk in a forest food web. Ecology. (2018) 99:1562–73. doi: 10.1002/ecy.2386
288. Kilpatrick AM, Dobson ADM, Levi T, Salkeld DJ, Swei A, Ginsberg HS, et al. Lyme disease ecology in a changing world: consensus, uncertainty and critical gaps for improving control. Philos Trans R Soc Lond B Biol Sci. (2017) 372:20160117. doi: 10.1098/rstb.2016.0117
289. Turney S, Gonzalez A, Millien V. The negative relationship between mammal host diversity and Lyme disease incidence strengthens through time. Ecology , (2014) 95:3244–50. doi: 10.1890/14-0980.1
290. Izac JR, Camire AC, Schuler EJA, Hatke AL, O'Bier NS, Oliver LD Jr, et al. Serologic evidence for the exposure of eastern coyotes ( Canis latrans ) in Pennsylvania to the tick-borne pathogens Borreliella burgdorferi and Anaplasma phagocytophilum. mSphere. (2020) 5:e00544–20. doi: 10.1128/mSphere.00544-20
291. Kugeler KJ, Jordan RA, Schulze TL, Griffith KS, Mead PS. Will culling white-tailed deer prevent lyme disease? Zoonoses Public Health. (2016) 63:337–45. doi: 10.1111/zph.12245
292. Vázquez M, Muehlenbein C, Cartter M, Hayes EB, Ertel S, Shapiro ED. Effectiveness of personal protective measures to prevent Lyme disease. Emerg Infect Dis. (2008) 14:210–6. doi: 10.3201/eid1402.070725
293. Richardson M, Khouja C, Sutcliffe K. Interventions to prevent Lyme disease in humans: a systematic review. Prev Med Rep. (2019) 13:16–22. doi: 10.1016/j.pmedr.2018.11.004
294. Fischhoff IR, Bowden SE, Keesing F, Ostfeld RS. Systematic review and meta-analysis of tick-borne disease risk factors in residential yards, neighborhoods, and beyond. BMC Infect Dis. (2019) 19:861. doi: 10.1186/s12879-019-4484-3
295. Keesing F, Ostfeld RS. The tick project: testing environmental methods of preventing tick-borne diseases. Trends Parasitol . (2018) 34:447–50. doi: 10.1016/j.pt.2018.03.005
296. Bisanzio D, Fernández MP, Martello E, Reithinger R, Diuk-Wasser MA. Current and future spatiotemporal patterns of Lyme disease reporting in the Northeastern United States. JAMA Netw Open. (2020) 3:e200319. doi: 10.1001/jamanetworkopen.2020.0319
297. Ostfeld RS, Brunner JL. Climate change and Ixodes tick-borne diseases of humans. Philos Trans R Soc Lond B Biol Sci. (2015) 370:20140051. doi: 10.1098/rstb.2014.0051
298. Schwan TG, Piesman J, Golde WT, Dolan MC, Rosa PA. Induction of an outer surface protein on Borrelia burgdorferi during tick feeding. Proc Natl Acad Sci USA. (1995) 92:2909–13. doi: 10.1073/pnas.92.7.2909
299. de Silva AM, Telford SR 3rd, Brunet LR, Barthold SW, Fikrig E. Borrelia burgdorferi OspA is an arthropod-specific transmission-blocking Lyme disease vaccine. J Exp Med. (1996) 183:271–5. doi: 10.1084/jem.183.1.271
300. Hanson MS, Edelman R. Progress and controversy surrounding vaccines against Lyme disease. Expert Rev Vaccines. (2003) 2:683–703. doi: 10.1586/14760584.2.5.683
301. Poland GA. Vaccines against Lyme disease: what happened and what lessons can we learn? Clin Infect Dis. (2011) 52(Suppl. 3):s253–8. doi: 10.1093/cid/ciq116
302. Embers ME, Narasimhan S. Vaccination against Lyme disease: past, present, and future. Front Cell Infect Microbiol. (2013) 3:6. doi: 10.3389/fcimb.2013.00006
303. Willyard C. Resurrecting the “yuppie vaccine.” Nat Med . (2014) 20:698–701. doi: 10.1038/nm0714-698
304. Shaffer L. Inner Workings: Lyme disease vaccines face familiar challenges, both societal and scientific. Proc Natl Acad Sci USA. (2019) 116:19214–7. doi: 10.1073/pnas.1913923116
305. Gomes-Solecki M, Arnaboldi PM, Backenson PB, Benach JL, Cooper CL, Dattwyler RJ, et al. Protective immunity and new vaccines for Lyme disease. Clin Infect Dis . (2019) 70:1768–73. doi: 10.1093/cid/ciz872
306. Comstedt P, Schüler W, Meinke A, Lundberg U. The novel Lyme borreliosis vaccine VLA15 shows broad protection against Borrelia species expressing six different OspA serotypes. PLoS ONE. (2017) 12:e0184357. doi: 10.1371/journal.pone.0184357
307. Alternative Schedule Study For VLA15, a Vaccine Candidate Against Lyme Borreliosis - Full Text View . ClinicalTrials.gov. Available online at: https://clinicaltrials.gov/ct2/show/NCT03970733?recrs=abdf&cond=Lyme+Disease&draw=3&rank=15 (accessed March 17, 2020).
308. Immunogenicity and Safety Study of a Vaccine Against Lyme Borreliosis, in Healthy Adults Aged 18 to 65 Years. Randomized, Controlled, Observer-blind Phase 2 Study. - Full Text View . ClinicalTrials.gov. Available online at: https://clinicaltrials.gov/ct2/show/NCT03769194 (accessed March 17, 2020).
309. Kamp HD, Swanson KA, Wei RR, Dhal PK, Dharanipragada R, Kern A, et al. Design of a broadly reactive Lyme disease vaccine. NPJ Vaccines. (2020) 5:33. doi: 10.1038/s41541-020-0183-8
310. Izac JR, Oliver LD Jr, Earnhart CG, Marconi RT. Identification of a defined linear epitope in the OspA protein of the Lyme disease spirochetes that elicits bactericidal antibody responses: implications for vaccine development. Vaccine. (2017) 35:3178–85. doi: 10.1016/j.vaccine.2017.04.079
311. Izac JR, Camire AC, Earnhart CG, Embers ME, Funk RA, Breitschwerdt EB, et al. Analysis of the antigenic determinants of the OspC protein of the Lyme disease spirochetes: evidence that the C10 motif is not immunodominant or required to elicit bactericidal antibody responses. Vaccine. (2019) 37:2401–7. doi: 10.1016/j.vaccine.2019.02.007
312. Izac JR, O'Bier NS, Oliver LD Jr, Camire AC, Earnhart CG, LeBlanc Rhodes DV, et al. Development and optimization of OspC chimeritope vaccinogens for Lyme disease. Vaccine . (2020) 38:1915–24. doi: 10.1016/j.vaccine.2020.01.027
313. O'Bier NS, Hatke AL, Camire AC, Marconi RT. Human and veterinary vaccines for Lyme disease. Curr Issues Mol Biol. (2020) 42:191–222. doi: 10.21775/cimb.042.191
314. Marconi RT, Garcia-Tapia D, Hoevers J, Honsberger N, King VL, Ritter D, et al. VANGUARD®crLyme: A next generation Lyme disease vaccine that prevents B. burgdorferi infection in dogs. Vaccine X. (2020) 6:100079. doi: 10.1016/j.jvacx.2020.100079
315. Marcinkiewicz AL, Lieknina I, Kotelovica S, Yang X, Kraiczy P, Pal U, et al. Eliminating factor H-binding activity of Borrelia burgdorferi CspZ combined with virus-like particle conjugation enhances its efficacy as a Lyme disease vaccine. Front Immunol. (2018) 9:181. doi: 10.3389/fimmu.2018.00181
316. Rego ROM, Trentelman JJA, Anguita J, Nijhof AM, Sprong H, Klempa B, et al. Counterattacking the tick bite: towards a rational design of anti-tick vaccines targeting pathogen transmission. Parasites Vectors. (2019) 12:229. doi: 10.1186/s13071-019-3468-x
317. Nelder MP, Russell CB, Sheehan NJ, Sander B, Moore S, Li Y, et al. Human pathogens associated with the blacklegged tick Ixodes scapularis: a systematic review. Parasit Vectors. (2016) 9:265. doi: 10.1186/s13071-016-1529-y
318. Crowder CD, Carolan HE, Rounds MA, Honig V, Mothes B, Haag H, et al. Prevalence of Borrelia miyamotoi in Ixodes ticks in Europe and the United States. Emerg Infect Dis. (2014) 20:1678–82. doi: 10.3201/eid2010.131583
319. Krause PJ, Narasimhan S, Wormser GP, Barbour AG, Platonov AE, Brancato J, et al. Borrelia miyamotoi sensu lato seroreactivity and seroprevalence in the northeastern United States. Emerg Infect Dis. (2014) 20:1183–90. doi: 10.3201/eid2007.131587
320. Prusinski MA, Kokas JE, Hukey KT, Kogut SJ, Lee J, Backenson PB. Prevalence of Borrelia burgdorferi (Spirochaetales: Spirochaetaceae), Anaplasma phagocytophilum (Rickettsiales: Anaplasmataceae), and Babesia microti (Piroplasmida: Babesiidae) in Ixodes scapularis (Acari: Ixodidae) collected from recreational lands in the Hudson Valley Region, New York State. J Med Entomol. (2014) 51:226–36. doi: 10.1603/ME13101
321. Gray EB, Herwaldt BL. Babesiosis Surveillance - United States, 2011-2015. MMWR Surveill Summ. (2019) 68:1–11. doi: 10.15585/mmwr.ss6806a1
322. Levin ML, Ross DE. Acquisition of different isolates of Anaplasma phagocytophilum by Ixodes scapularis from a model animal. Vector Borne Zoonotic Dis. (2004) 4:53–9. doi: 10.1089/153036604773082997
323. Pritt BS, Sloan LM, Johnson DKH, Munderloh UG, Paskewitz SM, McElroy KM, et al. Emergence of a new pathogenic Ehrlichia species, Wisconsin and Minnesota, 2009. N Engl J Med. (2011) 365:422–9. doi: 10.1056/NEJMoa1010493
324. Anderson JF, Armstrong PM. Prevalence and genetic characterization of Powassan virus strains infecting Ixodes scapularis in Connecticut. Am J Trop Med Hyg. (2012) 87:754–9. doi: 10.4269/ajtmh.2012.12-0294
325. Dupuis AP II, Peters RJ, Prusinski MA, Falco RC, Ostfeld RS, Kramer LD. Isolation of deer tick virus (Powassan virus, lineage II) from Ixodes scapularis and detection of antibody in vertebrate hosts sampled in the Hudson Valley, New York State. Parasit Vectors. (2013) 6:185. doi: 10.1186/1756-3305-6-185
326. Dai J, Wang P, Adusumilli S, Booth CJ, Narasimhan S, Anguita J, et al. Antibodies against a tick protein, Salp15, protect mice from the Lyme disease agent. Cell Host Microbe. (2009) 6:482–92. doi: 10.1016/j.chom.2009.10.006
327. Wikel SK. Host immunity to ticks. Ann Rev Entomol. (1996) 41:1–22. doi: 10.1146/annurev.en.41.010196.000245
328. Murfin KE, Fikrig E. Tick bioactive molecules as novel therapeutics: beyond vaccine targets. Front Cell Infect Microbiol. (2017) 7:222. doi: 10.3389/fcimb.2017.00222
329. Narasimhan S, Schuijt TJ, Abraham NM, Rajeevan N, Coumou J, Graham M, et al. Modulation of the tick gut milieu by a secreted tick protein favors Borrelia burgdorferi colonization. Nat Commun. (2017) 8:184. doi: 10.1038/s41467-017-00208-0
330. Murfin KE, Kleinbard R, Aydin M, Salazar SA, Fikrig E. Borrelia burgdorferi chemotaxis toward tick protein Salp12 contributes to acquisition. Ticks Tick Borne Dis. (2019) 10:1124–34. doi: 10.1016/j.ttbdis.2019.06.002
331. Narasimhan S, Booth CJ, DePonte K, Wu M-J, Liang X, Mohanty S, et al. Host-specific expression of Ixodes scapularis salivary genes. Ticks Tick Borne Dis. (2019) 10:386–97. doi: 10.1016/j.ttbdis.2018.12.001
332. Narasimhan S, Kurokawa C, Diktas H, Strank NO, Cerný J, Murfin K, et al. Ixodes scapularis saliva components that elicit responses associated with acquired tick-resistance. Ticks Tick Borne Dis. (2020) 11:101369. doi: 10.1016/j.ttbdis.2019.101369
333. Eisen L. Pathogen transmission in relation to duration of attachment by Ixodes scapularis ticks. Ticks Tick Borne Dis. (2018) 9:535–42. doi: 10.1016/j.ttbdis.2018.01.002
334. de la Fuente J, Kocan KM. Advances in the identification and characterization of protective antigens for recombinant vaccines against tick infestations. Exp Rev Vaccines. (2003) 2:583–93. doi: 10.1586/14760584.2.4.583
335. Carreón D, de la Lastra JMP, Almazán C, Canales M, Ruiz-Fons F, Boadella M, et al. Vaccination with BM86, subolesin and akirin protective antigens for the control of tick infestations in white tailed deer and red deer. Vaccine. (2012) 30:273–9. doi: 10.1016/j.vaccine.2011.10.099
336. Gortazar C, Diez-Delgado I, Barasona JA, Vicente J, De La Fuente J, Boadella M. The wild side of disease control at the wildlife-livestock-human interface: a review. Front Vet Sci. (2014) 1:27. doi: 10.3389/fvets.2014.00027
337. Molins CR, Sexton C, Young JW, Ashton LV, Pappert R, Beard CB, et al. Collection and characterization of samples for establishment of a serum repository for lyme disease diagnostic test development and evaluation. J Clin Microbiol. (2014) 52:3755–62. doi: 10.1128/JCM.01409-14
338. Marques A, Schwartz I, Wormser GP, Wang Y, Hornung RL, Demirkale CY, et al. Transcriptome assessment of erythema migrans skin lesions in patients with early Lyme disease reveals predominant interferon signaling. J Infect Dis. (2017) 217:158–67. doi: 10.1093/infdis/jix563
339. Johnson L, Shapiro M, Mankoff J, Johnson L, Shapiro M, Mankoff J. Removing the mask of average treatment effects in chronic Lyme disease research using big data and subgroup analysis. Healthc Pap. (2018) 6:124. doi: 10.3390/healthcare6040124
340. Johnson L, Shapiro M, Stricker RB, Vendrow J, Haddock J, Needell D. Antibiotic treatment response in chronic Lyme disease: why do some patients improve while others do not? Healthcare. (2020) 8:383. doi: 10.3390/healthcare8040383
341. Vilcarromero S, Nunez AM, Vivas K, Mahmood S, Russo J, Wellins A-M, et al. 1633. Human co-infection with Borrelia burgdorferi and Babesia microti Among High-Risk Hispanic/Latino Workers on Eastern Long Island, New York: A Preliminary Cross-Sectional Analysis in 2016. Open For Infect Dis . (2019) 6:S596. doi: 10.1093/ofid/ofz360.1497
342. Vilcarromero S, Nunez AM, Vivas K, Russo J, Mahmood S, Wellins A-M, et al. 1645. High seroprevalence and seroconversion rate of Borrelia burgdorferi infection among Hispanic/Latino Immigrant Workers in Eastern Suffolk County, New York: A Longitudinal-Based Study. Open For Infect Dis. (2019) 6:S600. doi: 10.1093/ofid/ofz360.1509
343. Collins SM. Kay Hagan Tick Act. 1657 . (2019). Available online at: https://www.congress.gov/bill/116th-congress/senate-bill/1657/text/is (accessed July 22, 2021).
344. Brodin P. Immune determinants of COVID-19 disease presentation and severity. Nat Med. (2021) 27:28–33. doi: 10.1038/s41591-020-01202-8
Keywords: Lyme disease, pathogenesis, diagnosis, treatment, prevention, field building, PTLD, vaccine
Citation: Bobe JR, Jutras BL, Horn EJ, Embers ME, Bailey A, Moritz RL, Zhang Y, Soloski MJ, Ostfeld RS, Marconi RT, Aucott J, Ma'ayan A, Keesing F, Lewis K, Ben Mamoun C, Rebman AW, McClune ME, Breitschwerdt EB, Reddy PJ, Maggi R, Yang F, Nemser B, Ozcan A, Garner O, Di Carlo D, Ballard Z, Joung H-A, Garcia-Romeu A, Griffiths RR, Baumgarth N and Fallon BA (2021) Recent Progress in Lyme Disease and Remaining Challenges. Front. Med. 8:666554. doi: 10.3389/fmed.2021.666554
Received: 10 February 2021; Accepted: 12 July 2021; Published: 18 August 2021.
Reviewed by:
Copyright © 2021 Bobe, Jutras, Horn, Embers, Bailey, Moritz, Zhang, Soloski, Ostfeld, Marconi, Aucott, Ma'ayan, Keesing, Lewis, Ben Mamoun, Rebman, McClune, Breitschwerdt, Reddy, Maggi, Yang, Nemser, Ozcan, Garner, Di Carlo, Ballard, Joung, Garcia-Romeu, Griffiths, Baumgarth and Fallon. This is an open-access article distributed under the terms of the Creative Commons Attribution License (CC BY) . The use, distribution or reproduction in other forums is permitted, provided the original author(s) and the copyright owner(s) are credited and that the original publication in this journal is cited, in accordance with accepted academic practice. No use, distribution or reproduction is permitted which does not comply with these terms.
*Correspondence: Jason R. Bobe, jason.bobe@mssm.edu
† ORCID: Jason R. Bobe orcid.org/0000-0003-1864-8609 Robert L. Moritz orcid.org/0000-0002-3216-9447 Panga Jaipal Reddy orcid.org/0000-0002-4481-3631
Disclaimer: All claims expressed in this article are solely those of the authors and do not necessarily represent those of their affiliated organizations, or those of the publisher, the editors and the reviewers. Any product that may be evaluated in this article or claim that may be made by its manufacturer is not guaranteed or endorsed by the publisher.
- Dermatology
- Gastroenterology
- Geriatric Medicine and Gerontology
- Gynecology and Obstetrics
- Heart and Vascular
- Neurology and Neurosurgery
- Ophthalmology
- Orthopaedics
- Otolaryngology–Head and Neck Surgery
- Physical Medicine and Rehabilitation
- Plastic and Reconstructive Surgery
- Psychiatry and Behavioral Sciences
- Pediatric Specialties
- Pediatric Diabetes and Endocrinology
- Pediatrics Florida
- Pediatric Gastroenterology and GI Surgery
- Pediatric Heart
- Pediatrics Maryland/DC
- Pediatric Neurology & Neurosurgery
- Pediatric Orthopaedics
- Physician Affiliations
- Health Care Technology
- High-Value Health Care
- Clinical Research Advancements
- Precision Medicine Excellence
- Patient Safety
PTLD: Recent Advancements in Long-Haul Lyme Disease Research
Some people get bitten by a deer tick that’s infected with Borrelia burgdorferi, develop a rash, are diagnosed with Lyme disease, take antibiotics, feel better, and get their life back to normal. Others aren’t so lucky. Diagnosed just as promptly, they take the exact same course of antibiotics. And then… they don’t get better. What’s happening?
John Aucott , M.D., an infectious diseases specialist and the founding Director/physician of the Johns Hopkins Lyme Disease Research Center, is a world authority on this “post-treatment Lyme disease” (PTLD), a condition he helped define. Its symptoms are persistent, and can include fatigue, pain, weakness, brain fog, and sleep disorders. He has studied and treated hundreds of patients with PTLD, who tell him things like, “I can’t get out of bed,” or “I can’t ride my bike,” or “I’m exhausted trying to get through the work day.”
In addition to feeling ill, frustrated, discouraged and even desperate for relief, these patients often must deal with a stigma – similar to that experienced by a brand-new group of patients from the pandemic, the Covid-19 ‘long-haulers,’ Aucott says. “The Covid long-haulers aren’t dying, they don’t have signs of extensive organ damage – but they have the exact same lingering, disabling symptoms that we have been pointing out for years in our PTLD patients.”
Aucott and his longtime colleague, scientist Mark Soloski , Ph.D., suspect that for the PTLD “long-haulers” – about 10 to 15 percent of people treated for acute (initial) Lyme disease – the disease process triggers persistent changes in the immune system and the autonomic nervous system. Their pioneering research program focuses on defining and characterizing changes in the underlying molecular mechanisms that cause and perpetuate these symptoms, with the hope of finding biomarkers to help diagnose and monitor this disease, and to discover new avenues of treatment.
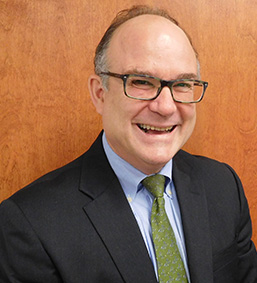
Their areas of active study include the gut microbiome. In work recently published online in mBio, Soloski, Aucott and colleagues at Hopkins, Northeastern University, and University of California-San Diego reported that PTLD patients have a distinct microbiome “signature,” or population of bacteria in their gastrointestinal tract compared to healthy controls and to an intensive care unit (ICU) control group. The scientists analyzed fecal samples from patients in the Hopkins Study of Lyme Immunology and Clinical Endpoints (SLICE), and compared them to the healthy control group and to the ICU control group – patients who were also on antibiotics.
Share Fast Facts
Johns Hopkins scientists have identified six symptom factors and three potentially clinically relevant subgroups among patients with post-treatment Lyme disease. Click to Tweet
“We found that the PTLD group had two distinct differences in their gut bacteria – an abundance of Blautia bacteria, and a decrease in Bacteroides,” says Soloski. “Bacteroides is interesting, because it produces GABA, an important neurotransmitter.”
Low levels of GABA can cause anxiety and depression. In turn, an excess of Blautia has been found in people with obesity, Alzheimer’s disease, and multiple sclerosis. The good news about the gut microbiome is that it can be altered by many factors, including diet, medication, and even fecal transplant. It may be that reintroducing healthy bacteria into the colon could significantly improve quality of life in these patients – a compelling idea that the investigators feel is worthy of further study.
The Metabolic Response
In another study, Soloski, Aucott and colleagues at Hopkins, the Centers for Disease Control, Colorado State University, and New York Medical College found significant metabolic differences in people with PTLD compared to other patients. This work was published in Clinical Infectious Diseases. “Basically, we found that the metabolome – all those small molecules our cells make and pour out into our blood, our signature of the metabolic activities of all the cells in the body – is perturbed in patients with Lyme disease.”
The scientists have identified a “fingerprint” of metabolites unique to patients with PTLD. This might one day lead to a blood test to determine which patients with Lyme disease are at risk of PTLD, and to help monitor the course of illness in PTLD patients.
Is there a genetic fingerprint?
Soloski and Aucott are looking for epigenetic changes – small mutations in the structure of DNA – among the 20,000 or so genes in the genome. This is big-data analysis that wouldn’t have been possible a few years ago. Computers and data experts sift countless pieces of evidence, like miners sluicing for gold, looking for valuable nuggets: patterns of gene expression, particularly in immune system genes and in messenger RNA genes.
Better Treatment for PTLD
“There is no FDA-approved treatment for PTLD,” says Aucott, “so we use drugs that the FDA has approved for other indications. Treatment is very patient specific, depending on the primary symptoms.” For example, fatigue often goes along with postural orthostatic tachycardia syndrome (POTS), a condition where the heartbeat skyrockets with a change of position – from sitting to standing, for example – that is treated by blood pressure-regulating drugs such as midodrine.
In exciting research recently published online in BMJ Open, Hopkins scientists Alison Rebman , Ting Yang, and Aucott, have identified six symptom factors and three potentially clinically relevant subgroups among patients with PTLD. The group’s findings may be an important step toward developing even more personalized and specific treatment plans.
Aucott has spent years working to raise physician awareness about PTLD, which “doesn’t fit into one specific disease silo” or subspecialty; in fact, the Center is the only one of its kind based in an academic department of medicine with a focus on PTLD.
New to the Center is rheumatologist John Miller , M.D., who brings expertise in joint ultrasound. “He is showing changes in our patients that are subtle,” particularly “tendinopathy-related causes of periarticular pain that nobody’s seen before, because joint ultrasound has never been done in these patients.” Miller has found enthesitis, inflammation where tendons insert into the joints that is also found in psoriatic arthritis and Reiter’s syndrome (reactive arthritis). His findings “may lead to looking at autoimmune-related drugs,” says Aucott, and the images “are opening up a whole new field of clinical inquiry. Nobody else is really doing this.”
To learn more about the Johns Hopkins Lyme Disease Research Center, visit hopkinslyme.org .
- About Johns Hopkins Medicine
- Contact Johns Hopkins Medicine
- Centers & Departments
- Maps & Directions
- Find a Doctor
- Patient Care
- Terms & Conditions of Use
- Privacy Statement
Connect with Johns Hopkins Medicine

Join Our Social Media Communities >
Clinical Connection
- Otolaryngology—Head and Neck Surgery
- Contact Johns Hopkins
© The Johns Hopkins University, The Johns Hopkins Hospital, and Johns Hopkins Health System. All rights reserved.

Privacy Policy and Disclaimer


Potential new treatment path for lasting Lyme disease symptoms
Tulane University researchers have identified a promising new approach to treating persistent neurological symptoms associated with Lyme disease, offering hope to patients who suffer from long-term effects of the bacterial infection, even after antibiotic treatment. Their results were published in Frontiers in Immunology .
Lyme disease, caused by the bacterium Borrelia burgdorferi and transmitted through tick bites, can lead to a range of symptoms, including those affecting the central and peripheral nervous systems. While antibiotics can effectively clear the infection in most cases, a subset of patients continues to experience symptoms such as memory loss, fatigue, and pain -- a condition often referred to as post-treatment Lyme disease syndrome.
Principal investigator Geetha Parthasarathy, PhD, an assistant professor of microbiology and immunology at the Tulane National Primate Research Center, has discovered that fibroblast growth factor receptor inhibitors, a type of drug previously studied in the context of cancer, can significantly reduce inflammation and cell death in brain and nerve tissue samples infected with Borrelia burgdorferi. This discovery suggests that targeting FGFR pathways may offer an exciting new therapeutic approach to addressing persistent neuroinflammation in patients with post-treatment Lyme disease syndrome.
"Our findings open the door to new research approaches that can help us support patients suffering from the lasting effects of Lyme disease," Parthasarathy said. "By focusing on the underlying inflammation that contributes to these symptoms, we hope to develop treatments that can improve the quality of life for those affected by this debilitating condition."
Researchers treated nerve tissue with live or inactivated Borrelia burgdorferi, followed by an application of FGFR inhibitors. Study results revealed a significant reduction in both inflammatory markers and of cell death.
While further research is needed to translate these findings into clinical treatments, the study represents an important step forward in understanding and potentially managing the complex aftermath of Lyme disease.
This study was funded by the Bay Area Lyme Foundation and supported with resources from the Tulane National Primate Research Center base grant of the National Institutes of Health, P51 OD011104.
- Lyme Disease
- Diseases and Conditions
- Alzheimer's
- Veterinary Medicine
- Lyme disease
- Stem cell treatments
- West Nile virus
- Ovarian cancer
- Chemotherapy
Story Source:
Materials provided by Tulane University . Note: Content may be edited for style and length.
Journal Reference :
- Geetha Parthasarathy. Fibroblast growth factor receptor inhibitors mitigate the neuropathogenicity of Borrelia burgdorferi or its remnants ex vivo . Frontiers in Immunology , 2024; 15 DOI: 10.3389/fimmu.2024.1327416
Cite This Page :
Explore More
- PFAS: US Drinking Water Contamination
- Chronic Pain Predicted Within 3 Days of Injury
- Electric Cars: Long-Lasting Renewable Batteries
- Wildfires Becoming Faster, More Dangerous
- Beyond Superheavy Element Livermorium
- Weight-Loss Drug May Cut Alzheimer's Risk
- Human Actions Cause Insect Color Change
- Better Brain-Machine Interfaces, Pacemakers
- Gut Bacterial Enzyme's Wide-Ranging Functions
- Tiny Electric Currents May Cut Skin Infections
Trending Topics
Strange & offbeat.
- Member Login

- About Lyme Disease
- Lyme Disease Symptoms
- Lyme Symptoms Checklist
- Chronic Lyme
- Lyme Disease Diagnosis
- Lyme Disease Tests
- Lyme Disease Treatment
- Children and Lyme
- Pets and Lyme
- About Ticks
- Types of Ticks
- Personal Protection
- Tick Removal
- Co-Infection Special Issue
- About CoInfections
- Ehrlichia and Anaplasma
- Mast Cell Activation Syndrome
- Alpha-gal Syndrome
- Other Co-Infections
- Join Online Support Groups
- Patient Resources
- Lyme Disease Events
- Lyme Disease Posters
- Financial Assistance
- Lyme Disease Book Reviews
- Healthcare Policy Resources
- Articles / Videos / Links
- International Resources
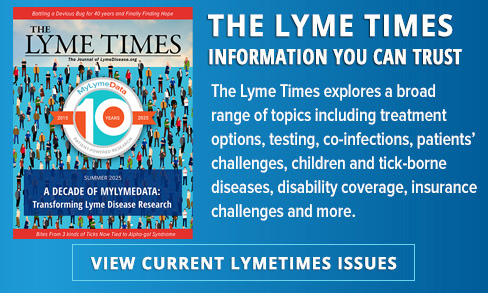
- View All LymeTimes Issues
- View Current Issue
- MCAS Special Issue
- Summer 2023 Issue
- COVID-19 Special Issue Issue
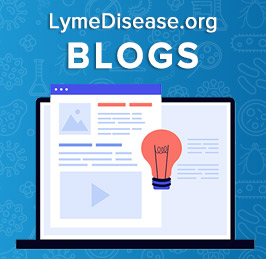
- MyLymeData Viz Blog
- Lyme Policy Wonk
- Touched By Lyme
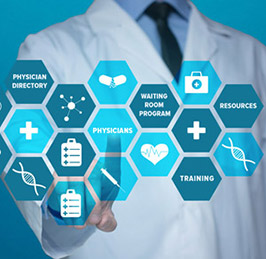
- Find A Lyme Disease Physician
- Register For Access
- Physician Programs
- Physician Directory - List Your Practice
- Physician Directory - Update Your Listing
- Physician Waiting Room Program
- Waiting Room Program For Patients
- Physicians Training & Resources
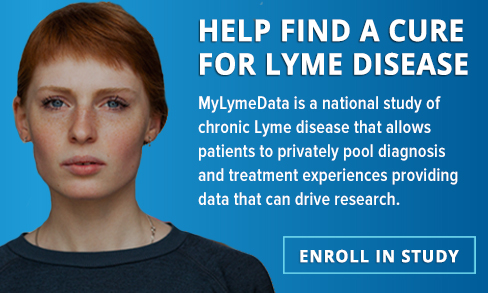
- MyLymeData 101
- About MyLymeData
- MyLymeData Highlights
- What Makes MyLymeData Different
- MyLymeData Videos
- MyLymeData Publications
- Previous Lyme Disease Studies
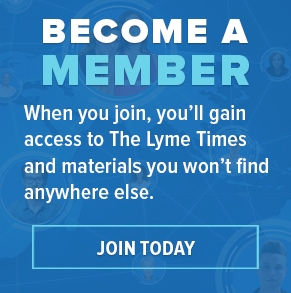
- Make A Donation
- Leave A Lasting Legacy
- Share LymeDisease.org
- About Membership
- Member Benefits
- Become A Member
- Membership Dashboard
- Political Action
- Patient Surveys
- The IDSA Lyme Guidelines
- Lyme Awareness Month
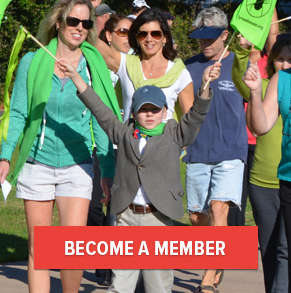
- Board Of Directors
- Advocacy and Public Policy
- Medical Journal Publications
- Healthcare Presentations
- Education and Outreach
- The Lyme Times
- Grassroots Advocacy
- Healthcare Policy
- Contact LymeDisease.org
- Become A Sponsor
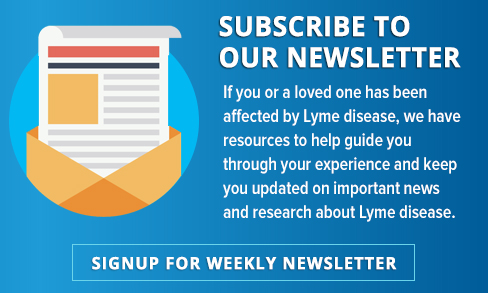
LymeDisease.org
“the state of lyme disease research in the united states”.
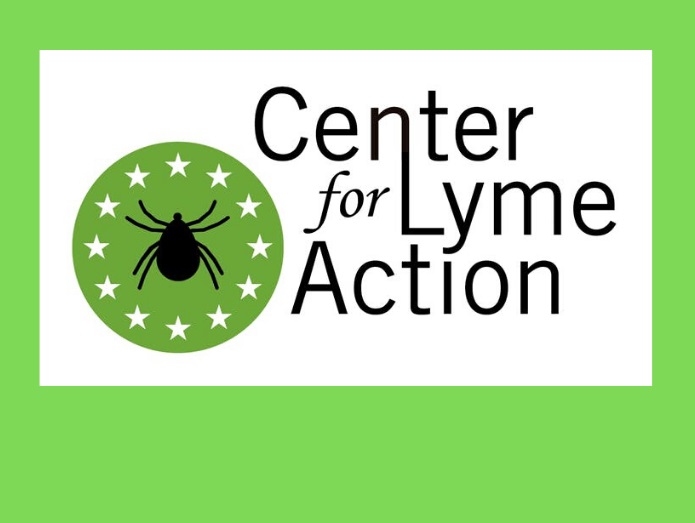
Center for Lyme Action, a nonprofit dedicated to growing federal funding for Lyme and tick-borne diseases, has published a research paper called “The State of Lyme Disease Research in the United States.”
With nearly half a million new cases of Lyme disease each year in the U.S., the paper chronicles the current published studies and makes recommendations for areas of federal investment.
“Lyme is a frustrating and debilitating disease, but it’s a solvable problem,” said Jeff Crater, co-founder of the Center for Lyme Action.
“Unfortunately, Lyme disease cases are rising, but with the right research funded to create new diagnostics and therapies we can make progress towards a cure.”
26 recommendations
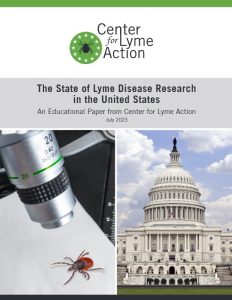
Key recommendations include:
- Increase research funding for the development of diagnostics that are sensitive and specific for the detection of Borrelia and other tick-borne infections.
- Increase research funding for alternative therapeutics to treat acute, late-stage, and persistent Lyme disease.
- Build a national biorepository of human samples for Lyme disease and other tick-borne illnesses supported by a network of qualified laboratories and physician clinics.
- Increase research and clinical studies funding to better understand the mechanisms for Borrelia persistence and its tolerance to antibiotics.
“While we need to improve our knowledge of Lyme and tick-borne diseases, fundamentally the diagnostics and therapies for Lyme disease have not changed very much in the last 20 years,” said Nicole Bell, primary author of the paper.
“In particular, Center for Lyme Action recommends significant investments in new diagnostics that will not only help physicians treat patients more effectively, but also can unlock innovative clinical trials for new treatments.”
Click here to read the paper.
SOURCE: Center for Lyme Action
We invite you to comment on our Facebook page.
Visit lymedisease.org facebook page.
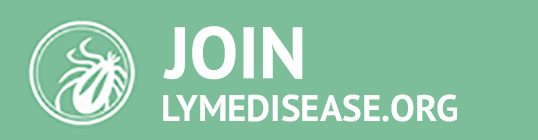
Newsletter Sign-up
News & blogs.
- Lyme Awareness
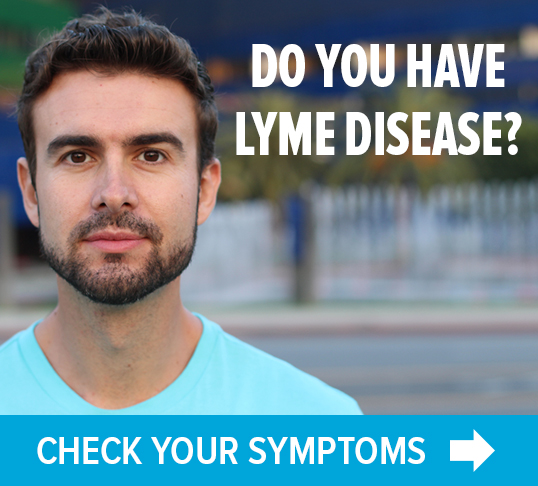
About LymeDisease.org
LymeDisease.org is a non-profit 501(c)(3) that serves the patient community through advocacy, education and research. Federal EIN-68-0214101
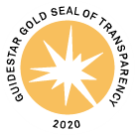
- Lyme Basics
- News & Blogs
- Focus – Opinions and Features
Support LymeDisease.org Become a member .
Comments? Questions? Ask your questions here .
Mailing Address
LymeDisease.org PO Box 16009 | San Diego, CA 92176
Recent Progress in Lyme Disease and Remaining Challenges
Affiliations.
- 1 Icahn School of Medicine at Mount Sinai, New York, NY, United States.
- 2 Department of Biochemistry, Fralin Life Sciences Institute, Virginia Tech, Blacksburg, VA, United States.
- 3 Lyme Disease Biobank, Portland, OR, United States.
- 4 Tulane University Health Sciences, New Orleans, LA, United States.
- 5 Institute for Systems Biology, Seattle, WA, United States.
- 6 State Key Laboratory for the Diagnosis and Treatment of Infectious Diseases, The First Affiliated Hospital, Zhejiang University School of Medicine, Hangzhou, China.
- 7 Division of Rheumatology, Department of Medicine, Lyme Disease Research Center, Johns Hopkins University School of Medicine, Baltimore, MD, United States.
- 8 Cary Institute of Ecosystem Studies, Millbrook, NY, United States.
- 9 Department of Microbiology and Immunology, Virginia Commonwealth University Medical Center, Richmond, VA, United States.
- 10 Bard College, Annandale, NY, United States.
- 11 Department of Biology, Northeastern University, Boston, MA, United States.
- 12 Yale University School of Medicine, New Haven, CT, United States.
- 13 Department of Clinical Sciences, Comparative Medicine Institute, College of Veterinary Medicine, North Carolina State University, Raleigh, NC, United States.
- 14 Department of Microbiology and Immunology, Indiana University School of Medicine, Indianapolis, IN, United States.
- 15 Steven & Alexandra Cohen Foundation, Stamford, CT, United States.
- 16 University of California, Los Angeles, Los Angeles, CA, United States.
- 17 Department of Psychiatry and Behavioral Sciences, Johns Hopkins University School of Medicine, Baltimore, MD, United States.
- 18 Center for Immunology and Infectious Diseases and the Department of Pathology, Microbiology & Immunology, School of Veterinary Medicine, University of California, Davis, Davis, CA, United States.
- 19 Columbia University Irving Medical Center, New York, NY, United States.
- PMID: 34485323
- PMCID: PMC8416313
- DOI: 10.3389/fmed.2021.666554
Lyme disease (also known as Lyme borreliosis) is the most common vector-borne disease in the United States with an estimated 476,000 cases per year. While historically, the long-term impact of Lyme disease on patients has been controversial, mounting evidence supports the idea that a substantial number of patients experience persistent symptoms following treatment. The research community has largely lacked the necessary funding to properly advance the scientific and clinical understanding of the disease, or to develop and evaluate innovative approaches for prevention, diagnosis, and treatment. Given the many outstanding questions raised into the diagnosis, clinical presentation and treatment of Lyme disease, and the underlying molecular mechanisms that trigger persistent disease, there is an urgent need for more support. This review article summarizes progress over the past 5 years in our understanding of Lyme and tick-borne diseases in the United States and highlights remaining challenges.
Keywords: Lyme disease; PTLD; diagnosis; field building; pathogenesis; prevention; treatment; vaccine.
Copyright © 2021 Bobe, Jutras, Horn, Embers, Bailey, Moritz, Zhang, Soloski, Ostfeld, Marconi, Aucott, Ma'ayan, Keesing, Lewis, Ben Mamoun, Rebman, McClune, Breitschwerdt, Reddy, Maggi, Yang, Nemser, Ozcan, Garner, Di Carlo, Ballard, Joung, Garcia-Romeu, Griffiths, Baumgarth and Fallon.
Publication types
Grants and funding.
- R01 AI083640/AI/NIAID NIH HHS/United States
- R01 AI157007/AI/NIAID NIH HHS/United States
- UL1 TR001863/TR/NCATS NIH HHS/United States
- P51 OD011104/OD/NIH HHS/United States
- R21 AI142302/AI/NIAID NIH HHS/United States
- R01 AI152235/AI/NIAID NIH HHS/United States
- R21 AI133335/AI/NIAID NIH HHS/United States
- Search Menu
- Sign in through your institution
- Advance articles
- Editor's Choice
- Supplement Archive
- Cover Archive
- IDSA Guidelines
- IDSA Journals
- The Journal of Infectious Diseases
- Open Forum Infectious Diseases
- Photo Quizzes
- State-of-the-Art Reviews
- Voices of ID
- Author Guidelines
- Open Access
- Why Publish
- IDSA Journals Calls for Papers
- Advertising and Corporate Services
- Advertising
- Journals Career Network
- Reprints and ePrints
- Sponsored Supplements
- Branded Books
- About Clinical Infectious Diseases
- About the Infectious Diseases Society of America
- About the HIV Medicine Association
- IDSA COI Policy
- Editorial Board
- Self-Archiving Policy
- For Reviewers
- For Press Offices
- Journals on Oxford Academic
- Books on Oxford Academic
Article Contents
Biology of the organism, epidemiology, clinical manifestations, congenital lyme disease, prevention of lyme disease, lyme anxiety.
- < Previous
Lyme Disease
- Article contents
- Figures & tables
- Supplementary Data
Eugene D. Shapiro, Michael A. Gerber, Lyme Disease, Clinical Infectious Diseases , Volume 31, Issue 2, August 2000, Pages 533–542, https://doi.org/10.1086/313982
- Permissions Icon Permissions
Lyme disease, which is caused by Borrelia burgdorferi and transmitted in the United States primarily by Ixodes scapularis (the deer tick), is the most common vectorborne disease in the United States. Its most frequent manifestation, a characteristic, expanding annular rash (ery-thema migrans), sometimes accompanied by myalgia, arthralgia, and malaise, occurs in nearly 90% of persons with symptomatic infection. Other manifestations of Lyme disease include seventh cranial nerve palsy, aseptic meningitis, and arthritis. Extensive coverage in the press about the serious effects of Lyme disease has led to widespread anxiety about this illness that is far out of proportion to the actual morbidity that it causes. This problem is exacerbated by the frequent use of serological tests to eliminate the possible diagnosis of Lyme disease in persons with only nonspecific symptoms (such as arthralgia or fatigue) who have a very low probability that Lyme disease is the cause of their symptoms. Consequently, misdiagnosis is frequent and is the most common cause of failure of treatment. The prognosis for most persons with Lyme disease is excellent.
Lyme disease, which is caused by the spirochete Borrelia burgdorferi , is the most common vectorborne illness in the United States. Although we now know that a form of the illness was recognized in Scandinavia in the early 1900s, modern awareness of Lyme disease began when a cluster of children with “juvenile rheumatoid arthritis” on one small street was reported by their parents in the mid-1970s. Investigation of this unexplained “epi-demic” of arthritis led to the description of Lyme arthritis in 1976 and, ultimately, to the discovery of its bacterial etiology [ 1–3 ]. Both the reported incidence of the disease and its geographic range have increased dramatically in recent years [ 4 ]. This has been accompanied by an increase in publicity about the illness in the press and on the Internet, which at times has been accompanied by near-hysteria about both risks and complications of Lyme disease. This publicity, combined with a high frequency of misdiagnoses for people with nonspecific symptoms due to other causes, has resulted in anxiety about Lyme disease (among both patients and physicians) that is out of proportion to the actual morbidity that it causes.
B. burgdorferi is a gram-negative organism that is 10–30 µm long and 0.2–0.25 µm wide. It grows best at 30°C–34°C in a microaerophilic atmosphere, dividing every 8–12 h during log-phase growth [ 5 , 6 ]. It is routinely grown in liquid cultures in BSK II medium. Ultrastructurally, B. burgdorferi resembles other spirochetes in the genus Borrelia , with 7–11 periplasmic flagella that are attached subterminally to the protoplasmic cylinder and overlap in the center of the cell. The main structural component of the flagella is flagellin, a 41-kDa protein. The outer membrane contains an abundance of outer surface proteins (Osps) anchored to the outer membrane via lipid moieties at their amino termini (some may also extend to the cytoplasmic membrane). These include OspA, OspB, and OspC (lipoproteins of ∼132, 34, and 24 kDa, respectively). Although the role these Osps play during infection is not known, different Osp genes are expressed at different times during transmission from the tick vector to the mammalian host. Another antigen of B. burgdorferi is the 60-kDa antigen, which has been termed “common antigen” and belongs to the heat-shock protein family. Unlike other spirochetes, B. burgdorferi has linear chromosomes and 4–119 linear and circular plasmids. The genes that encode the major Osps of B. burgdorferi are located on plasmids.
Recent phylogenetic studies based on relatedness of DNA have subdivided the organism that causes Lyme disease, B. burgdorferi sensu lato, into multiple genospecies: B. burgdorferi sensu stricto, Borrelia garinii , and Borrelia afzelii. In the United States, a number of genospecies of B. burgdorferi sensu lato have been isolated from animals and from ticks, but only B. burgdorferi sensu stricto has been isolated from humans. In contrast, there is substantial antigenic variability among human isolates of B. burgdorferi in Europe.
Lyme disease has been reported from 49 states (although in many states there is no endemic disease—1the reports are are either of imported cases or of Lyme-like disease caused by other agents) and from >50 countries. In the United States, most of the cases of Lyme disease occur in southern New England, the eastern parts of the middle Atlantic states, and the upper Midwest. There is a smaller endemic focus of Lyme disease along the northern Pacific coast. In Europe, most cases occur in the Scandinavian countries and in central Europe (especially Germany, Austria, and Switzerland).
Estimates of the incidence of Lyme disease in the United States are complicated by passive reporting systems and the high frequency of misdiagnosis of this illness. More than 100,000 cases of Lyme disease have been reported to the Centers for Disease Control and Prevention in the past 10 years. In areas of endemicity, the reported annual incidence ranges from 20 to slightly more than 100 cases per 100,000 people, although this figure may be as high as 1000 cases per 100,000 people in areas of hyperendemicity such as Lyme, Connecticut. Connecticut has the highest reported incidence of Lyme disease: 105 per 100,000 persons in 1998. The reported incidence is highest among children aged 5–10 years, which in most areas is almost twice as high as the incidence among older children and adults.
Lyme disease is a zoonosis. It is acquired by the transmission of B. burgdorferi to humans through the bite of an infected tick of the Ixodes species. In the eastern and midwestern United States, the vector is Ixodes scapularis (formerly known as Ixodes dammini ), the black-legged tick that is commonly known as the deer tick. In the western United States, the disease is transmitted by a closely related tick, I. pacificus —the western black-legged tick. The tick has a 2-year, 3-stage life cycle and feeds once during each stage [ 7 ]. The larvae hatch in the summer and are usually uninfected with B. burgdorferi because transovarial transmission of the spirochete is rare. The tick may become infected at any stage of its life cycle by feeding on a host, usually a small mammal (in particular the white-footed mouse, Peromyscus leucopus ), that is a natural reservoir for B. burgdorferi . Larvae emerge the following spring in the nymphal stage. The nymphal-stage tick is most likely to transmit the infection, presumably because it is so small it is difficult to identify the bite and to remove the tick in a timely manner. In addition, because it is small it becomes engorged more quickly than do adult ticks (engorgement is necessary before the organism can be transmitted). Moreover, nymphs are prevalent during spring and summer, when humans frequently enter habitats in which ticks thrive. The nymphs molt to adults in the fall. The females lay their eggs the following spring before they die and the 2-year life cycle begins again.
Several factors have been associated with the risk of transmission of B. burgdorferi from ticks to humans. First, the proportion of infected ticks varies both by geographic area and by the stage of the tick in its life cycle. In areas of endemicity in the northeastern and midwestern United States, ∼10%–20% of nymphal-stage I. scapularis and ∼30%–40% of adult ticks are infected with B. burgdorferi . There are small foci in which the rate of infection of adult deer ticks is 80% or even higher. By contrast, I. pacificus often feeds on lizards, which are a poor reservoir for B. burgdorferi . Consequently, only 1%–3% of these ticks, even in the nymphal and adult stages, are infected with B. burgdorferi .
Second, the risk of transmission of B. burgdorferi from infected Ixodes ticks is related to the duration of feeding. It takes hours for the mouthparts of ticks to implant fully in the host and much longer (days) for the tick to become fully engorged. The bites of these ticks are painless, in part because they secrete enzymes (such as bradykininases) that break down mediators of inflammation. Experiments with animals have shown that both nymphal and adult ticks must feed for ∼48 h or longer before the risk of transmission of B. burgdorferi becomes substantial [ 8 , 9 ]. Most persons who recognize that they have been bitten will remove the tick before the transmission of B. burgdorferi can occur [ 10 ]. Consequently, a history of a deer tick bite should serve mainly as a marker that the person is potentially exposed, because the risk of Lyme disease probably is greater from an unrecognized bite; unrecognized ticks are more likely to feed long enough to allow them to transmit the organism. People with greater occupational, recreational, or resi-den-tial exposure to either tick-infested woods or fields near woods (the preferred habitats of ticks) in areas of endemicity are at greater risk of developing Lyme disease. Other potential risk factors, such as race or sex, have not been studied adequately.
The clinical manifestations of Lyme disease depend on the stage of the illness—early localized disease; early disseminated disease; or late disease [ 11 , 12 ]. The most common manifestation of early localized Lyme disease, erythema migrans ( figure 1 ), appears at the site of a tick bite 3–30 days (but typically within 7–10 days) after the bite. It is recognized in ∼90% of patients who have objective evidence of B. burgdorferi infection [ 12 , 13 ]. Erythema migrans begins as a red macule or papule and, if untreated, expands for days to weeks to form a large, annular, erythematous lesion that is as much as 70 cm in diameter (median, 15 cm). This rash may be uniformly erythematous or it may appear as a target lesion with variable degrees of central clearing. It can vary greatly in shape and occasionally may have vesicular or necrotic areas in the center. Erythema migrans is usually asymptomatic but may be pruritic or painful, and it may be accompanied by systemic clinical features such as fever, malaise, headache, myalgia, or arthralgia. Other clinical findings that may be associated with erythema migrans include headache, regional lymphadenopathy, and a stiff neck. Conjunctivitis has also been described in patients with erythema migrans, but in recent studies of culture-positive patients with erythema migrans, conjunctivitis was rare [ 14 ].
Erythema migrans
Patients with early Lyme disease may also present with an illness similar to those caused by viruses (e.g., with fever, malaise, and myalgia) without erythema migrans. However, because both the sensitivity and specificity of serological tests for early Lyme disease are poor and because viral illness—like symptoms from causes other than Lyme disease are common, it is difficult to confirm a diagnosis of Lyme disease in patients with nonspecific, acute symptoms. Although the frequency of this manifestation of Lyme disease is not known, information from clinical trials of Lyme vaccine (in which subjects had routine follow-up serology done by a reference laboratory) suggests that it is uncommon.
The most common manifestation of early disseminated Lyme disease is multiple erythema migrans. The secondary skin lesions, which usually appear 3–5 weeks after the tick bite, consist of multiple annular erythematous lesions similar to, but usually smaller than, the primary lesion. Other common manifestations of early disseminated Lyme disease are cranial nerve palsies, especially facial nerve palsy, and meningitis. Although rare, carditis, usually manifested by fluctuating degrees of atrioventricular block, may also occur at this stage. The carditis may be associated with dizziness, palpitations, dyspnea, chest pain, or syncope. Systemic symptoms such as myalgia, arthralgia, headache, and fatigue are common in the early disseminated stage. Eye involvement (e.g., uveitis, iritis, and optic neuritis) has also been reported in this stage of Lyme disease. However, because B. burgdorferi has only rarely been identified in these disorders, the role of B. burgdorferi in their pathogenesis is uncertain.
The most common manifestation of late Lyme disease in the United States is arthritis, which is usually monoarticular or oligoarticular and affects the large joints, particularly the knee. The arthritis occurs weeks to months after the initial infection. Although the joint typically is swollen and tender, the intense pain associated with a septic arthritis usually is not present. About 10% of adults and fewer than 5% of children with Lyme arthritis develop inflammatory joint disease that does not respond to antimicrobial agents and typically affects 1 knee for months to years. Because of the increased frequency of certain HLA-DR4 alleles in these patients, an autoimmune mechanism has been proposed [ 15–17 ]. Encephalitis, encephalopathy, and polyneuropathy are also manifestations of late Lyme disease, but these manifestations are rare [ 18 , 19 ].
Additional episodes of erythema migrans have been reported in patients who received appropriate antimicrobial therapy for an initial episode of erythema migrans. It has been assumed that prompt initiation of antimicrobial therapy for early Lyme disease can depress the humoral immune response to B. burgdorferi , thereby leaving such patients susceptible to reinfection from new infection with B. burgdorferi . However, although it is much less common in this setting, even patients with serum antibodies to B. burgdorferi after the initial episode occasionally may be susceptible to subsequent infection.
The clinical manifestations of Lyme disease also may depend on which genospecies of B. burgdorferi is causing the infection. The differences in genospecies found in Europe and in North America may account for differences in the frequencies of certain manifestations of Lyme disease in these areas. For example, neurological manifestations of Lyme disease are more common in Europe, whereas rheumatological manifestations are more common in North America. In addition, certain skin and soft-tissue manifestations of Lyme disease, such as acrodermatitis chronica atrophicans and lymphocytomas, are usually caused by B. afzelii or B. garinii and, therefore, are seen in Europe but are extremely rare in the United States.
In the southern United Sates, a rash that resembles erythema migrans has been associated with the bite of Amblyomma americanum (the Lone Star tick). A new presumptive species, Borrelia lonestarii sp. nov., has been isolated from A. americanum ticks and may be the cause of this erythema migrans—like rash.
Ixodes ticks may transmit several other pathogens in addition to B. burgdorferi that are capable of causing human disease, including Babesia, Ehrlichia , and viruses [ 20 , 21 ]. These agents may be transmitted either separately or simultaneously with B. burgdorferi . Coinfection may alter both the clinical presentation and the response to treatment of patients with Lyme disease, although the frequency with which coinfection occurs is uncertain and its impact on the clinical presentation and response to treatment is not well characterized.
Much of the initial information about the potential for transplacental infection with Lyme disease was alarming [ 22 ]. However, this information came from a small number of case reports, most of which involved women with unrecognized and untreated Lyme disease during their pregnancy. There is also considerable skepticism about these cases, because none had evidence of inflammation and there was no consistent pattern of disease. In addition, although spirochetes compatible with B. burgdorferi were seen in pathological specimens, B. burgdorferi was never isolated in culture.
Several studies that assessed the potential link between Lyme disease during pregnancy and congenital infection with B. burgdorferi subsequently have been conducted [ 23–26 ]. In none of these studies was there a consistent pattern of disease or any clearly documented infections of either the fetus or the infant with B. burgdorferi . In addition, the obstetrical outcomes were similar among women who had documented Lyme disease during their pregnancies and those who did not. Moreover, in a survey of 162 child neurologists practicing in areas in which Lyme disease is endemic, the investigators were unable to identify any well-documented cases of prenatally acquired neuroborreliosis [ 27 ].
Thus, although there has been a temporal relationship between Lyme disease during pregnancy and adverse outcomes, a causal relationship has not been established [ 22 ]. There is no evidence of increased risk of abnormal outcomes with Lyme disease during pregnancy. Transmission of Lyme disease via breast-feeding has not been documented.
The clinical manifestations of Lyme disease, other than ery-thema migrans, are not specific. The monoarticular or pauciarticular arthritis may mimic either an acute septic joint or other causes of arthritis, such as juvenile rheumatoid arthritis or poststreptococcal arthritis in children or osteoarthritis in adults. Clinically, seventh cranial nerve palsy due to Lyme disease is indistinguishable from Bell's palsy, and Lyme meningitis may mimic meningitis due to an enterovirus. Even the diagnosis of erythema migrans may be difficult, because the rash initially may be confused with tinea, nummular eczema, granuloma annulare, an insect bite, or cellulitis. However, the relatively rapid expansion of erythema migrans helps to distinguish it from these other conditions. For this reason, if there is uncertainty about the diagnosis of the rash, observation for 1–3 days without treatment may be reasonable, especially if the patient is otherwise asymptomatic.
Isolation of B. burgdorferi from a symptomatic patient should be considered diagnostic of Lyme disease. Although B. burgdorferi has been isolated from blood, skin biopsies, cerebrospinal fluid, myocardial biopsies, and the synovium of patients with Lyme disease, the medium in which B. burgdorferi is cultured is expensive, it can take as long as 6 weeks for the bacteria to grow in culture, and the sensitivity of culture is only fair, except in research laboratories. Furthermore, it usually is necessary for patients to undergo an invasive procedure, such as a biopsy or a lumbar puncture, to obtain appropriate tissue or fluid for culture. B. burgdorferi has been identified with silver stains (Warthin-Starry or modified Dieterle's) and with immunohistochemical stains (with monoclonal or polyclonal antibodies) in skin, synovial, and myocardial biopsies. However, B. burgdorferi can be confused with normal tissue structures or it can be missed because it is often present in low concentrations.
Tests that use the polymerase chain reaction to identify the organism (particularly those done under carefully controlled conditions in research laboratories) may be helpful; however, the test may yield positive results even after all organisms have been killed, because DNA from the organism may persist for some time after the bacteria are no longer viable [ 28 ]. In addition, there is a risk of false-positive polymerase chain reaction tests, especially when they are done in commercial laboratories [ 29 ].
Attempts have been made to develop antigen-based diagnostic tests, but there are no convincing data that any test is accurate. All of these tests (including tests for antigen in the urine) should be considered experimental until additional studies confirm their validity and reproducibility. None of the tests for antigens of B. burgdorferi that have been adequately evaluated are sufficiently sensitive and specific to be clinically useful. Consequently, the confirmation of Lyme disease (if erythema migrans is not present) usually is based on the demonstration of antibodies to B. burgdorferi in the patient's serum.
The normal antibody response to infection with B. burgdorferi in untreated patients is well described [ 30 ]. Specific IgM antibodies appear first, usually 3–4 weeks after the infection begins. These antibodies peak after 6–8 weeks and subsequently decline, although a prolonged elevation of IgM antibodies sometimes occurs despite effective antimicrobial treatment [ 31 ]. Consequently, the results of tests for specific IgM antibodies should not be used as the sole indicator of the recency of an infection. Specific IgG antibodies usually appear 6–8 weeks after the onset of the infection. These antibodies peak in 4–6 months. The concentration of IgG antibodies to B. burgdorferi may decline after treatment, but even after the patient is clinically cured, these antibodies usually remain detectable for many years [ 32 ].
Because the immunofluorescent antibody test requires subjective interpretation and is time-consuming to perform, it has largely been replaced by ELISAs for the detection of antibodies against B. burgdorferi . The ELISA method sometimes produces false-positive results because of cross-reactive antibodies from patients with other spirochetal infections (e.g., syphilis, lepto-spirosis, or relapsing fever), with viral infections (e.g., varicella), or with certain autoimmune diseases (e.g., systemic lupus ery-the-ma-tosus), as well as because antibodies directed against spirochetes that are part of the normal oral flora may cross-react with antigens of B. burgdorferi .
Immunoblots (Western blots) for serum antibodies to B. burgdorferi are also used as a diagnostic test for Lyme disease. Although some investigators have suggested that the immunoblot is both more sensitive and more specific than the ELISA, there is still some debate about its interpretation. The immunoblot is most useful to validate a positive or equivocal ELISA result, especially for patients with a low clinical likelihood of having Lyme disease. For serological testing for Lyme disease, it is recommended that a quantitative test (such as an ELISA) be done and, if results are either positive or equivocal, that a Western immunoblot be done to confirm the specificity of the result [ 33 ].
An immunoblot may be necessary for diagnosis of persons who have received the Lyme vaccine. Because the ELISA uses whole-cell B. burgdorferi , this test may yield positive results for vaccinees, even though the vaccine induces antibodies only to the OspA protein of B. burgdorferi [ 34 ]. The interpretation of the Western immunoblot should not be affected by immunization, because the vaccine should induce antibodies only to the OspA protein. Immunoblot testing for non-OspA seroreactivity is critical for establishing or for excluding the diagnosis for patients suspected of having Lyme disease who have received the recombinant OspA vaccine. However, the predictive values of the interpretations of the results of tests with Western immunoblot kits that are used by most large commercial laboratories has not been well established.
One reason for the poor sensitivity of serological tests for Lyme disease is that erythema migrans, which is the clinical finding that usually brings patients to medical attention, normally develops within 2–3 weeks of the onset of the infection with B. burgdorferi . Antibodies to B. burgdorferi often are not detected at this early stage of the disease. The antibody response to B. burgdorferi also may be abrogated in patients with early Lyme disease who are treated promptly with an effective antimicrobial agent; these patients may never develop antibodies to B. burgdorferi , at least not as a result of that exposure.
Both the accuracy and the reproducibility of currently available serological tests, especially widely used commercially produced kits, are poor [ 35–39 ]. Use of these commercial diagnostic test kits for Lyme disease results in a high rate of misdiagnosis. Moreover, as with any diagnostic test, the predictive value of the test depends primarily on the probability that the patient has Lyme disease, which is determined on the basis of the patient's clinical and epidemiological history and physical examination (the pretest probability of Lyme disease). Use of serological tests to rule out Lyme disease in patients with a low probability of the illness will result in a high rate of false-positive tests [ 40 ]. With few exceptions, the probability that a patient has Lyme disease will be very low in areas in which Lyme disease is rare. Even in areas with a high prevalence of Lyme disease, patients with only nonspecific signs and symptoms, such as fatigue, headache, and arthralgia, are not likely to have Lyme disease [ 40 , 41 ]. The vast majority of positive serological tests for such patients are false-positive results—although such nonspecific symptoms are common in patients with Lyme disease, they are almost always accompanied by more specific objective findings, such as erythema migrans or arthritis. Even when more accurate tests done by reference laboratories are available, clinicians should order serological tests for Lyme disease selectively, reserving them for patients from populations with a relatively high prevalence of Lyme disease who have specific clinical findings that are suggestive of Lyme disease, so that the predictive value of a positive test is high [ 42 ].
Although demonstration of the intrathecal production of antibodies has been suggested as a means of confirming infection of the CNS with B. burgdorferi [ 43 ], interpretation of antibody tests of cerebrospinal fluid is complex. Consultation with experts is recommended to assist in the interpretation of such tests.
Table 1 shows recommended treatments for Lyme disease [ 44 , 45 ].
Recommended treatment of Lyme disease.
Early localized disease. Either doxycycline or amoxicillin is the drug of choice for patients aged ≥8 years. A substantial proportion of patients who take doxycycline develop a rash in sun-exposed areas; thus, precautions to avoid exposure to the sun (e.g., use of sunscreens) should be taken if doxycycline is used. Amoxicillin is recommended for patients aged <8 years (although a standard course of treatment with doxycycline is unlikely to stain the teeth of young children [ 46 ]), for those who cannot tolerate doxycycline, and for pregnant women. For patients aged <8 years who are allergic to penicillin (and for adults who cannot take either amoxicillin or doxycycline), alternative drugs are cefuroxime axetil (500 mg twice daily or, for children, 30 mg/kg/day divided twice daily), erythromycin, or azithromycin, although erythromycin and azithromycin may be less effective. Most experts recommend a 14- to 21-day course of therapy for early localized Lyme disease, although it is likely that shorter courses also are effective. Treatment of erythema migrans almost always prevents development of the later stages of Lyme disease. The usual clinical response to therapy is prompt, and erythema migrans resolves within several days of initiating therapy. Nonspecific symptoms (such as fatigue, arthralgia, or myalgia), which may persist for several weeks even in successfully treated patients, should not be regarded as an indication for additional treatment with antimicrobials. Patients with nonspecific symptoms should be treated symptomatically (e.g., with nonsteroidal anti-inflammatory agents). Occasionally, a Jarisch-Herxheimer reaction (usually marked by an elevated temperature and worsening myalgia) develops shortly after antimicrobial treatment is begun. Such reactions usually last for only 1–2 days. Antimicrobial treatment should not be discontinued, and patients may benefit from therapy with nonsteroidal anti-inflammatory agents.
Early disseminated and late disease. Multiple erythema migrans and arthritis should be treated with orally administered antimicrobial agents [ 44 , 45 , 47 , 48 ]. If peripheral facial nerve palsy is the only neurological manifestation of Lyme disease, the patient can be treated with an oral regimen of antimicrobials for 14–21 days. Corticosteroid therapy appears to be of no benefit. If the facial nerve palsy is accompanied by clinical evidence of CNS involvement (e.g., severe headache, nuchal rigidity), a lumbar puncture should be done. If there is pleocytosis, parenterally administered antimicrobials should be prescribed. The primary purpose of antimicrobial therapy for facial nerve palsy is to prevent progression to a late stage of Lyme disease; antimicrobial therapy apparently has little effect on the resolution of the palsy. Whether a patient is given antimicrobial therapy or not, the outcome of a facial nerve palsy due to Lyme disease is usually excellent, with complete resolution within 4–6 weeks. However, varying degrees of paralysis may persist in a small proportion of patients. Meningitis should be treated with parenterally administered antimicrobial agents. Mild carditis can be treated with the same oral regimens used for early localized disease, whereas severe carditis should be treated with antimicrobials administered parenterally.
A number of different treatment regimens have been used for Lyme arthritis, but none of them has been convincingly demonstrated to be superior. Lyme arthritis initially should be treated with orally administered antimicrobials for 28 days. If objective evidence of synovitis persists or recurs at least 2 months after treatment is initiated, a single course of parenteral antimicrobials can be administered for 14–28 days, although some experts would administer a second course of antimicrobial treatment orally before administering a parenteral agent. Neurological manifestations of late Lyme disease (e.g., encephalitis, encephalopathy, or polyneuropathy) should be treated with antimicrobials administered parenterally.
The optimal duration of antimicrobial therapy for early localized, early disseminated, or late Lyme disease has not been clearly established. However, there is no evidence that patients with any manifestation of Lyme disease benefit from either prolonged or repeated courses of antimicrobial therapy. A single course of antimicrobial therapy of >4 weeks' duration is not indicated. Lyme disease, like other infections, may trigger a fibromyalgia syndrome that does not respond to additional courses of antimicrobials but may improve with symptomatic therapy.
Prevention of tick bites. In residential areas of endemicity, clearing brush and trees, removing leaf litter and woodpiles, and keeping grass mowed may reduce exposure to ticks [ 49 ]. Application of pesticides to residential properties is effective in suppressing populations of ticks but may be harmful both to other wildlife and to people [ 50 ]. Erecting fences to exclude deer from residential yards and maintaining pets free of ticks also may reduce exposure to ticks [ 51 ].
Habitats that are heavily infested with ticks, such as wooded areas, should be avoided if possible. If not possible, exposure can be decreased by using wide trails, not straying off the trail, and not sitting on the ground. Preferred dress is light-colored clothing (to make recognition and removal of ticks easier), long sleeves that are tight at the wrists, and long pants that are tucked into light-colored socks. A hat should be worn in densely wooded areas.
Tick and insect repellents that contain n,n -diethylmetatoluamide (deet) applied to the skin provide additional protection but require reapplication every 1–2 h for maximum effectiveness. Serious neurological complications in children from either frequent or excessive application of deet-containing repellents have been reported, but they are rare and the risk is low when these products are used according to instructions on their labels [ 52–54 ]. Use of products with deet concentrations of >30% are not necessary and increase the risk of adverse effects. Deet should be applied sparingly only to exposed skin, but not to a child's face, hands, or skin that is either irritated or abraded. After the child returns indoors, skin that was treated should be washed with soap and water. Permethrin (a synthetic pyrethroid) is available in a spray for application to clothing only and is particularly effective because it kills ticks on contact.
Because studies indicate that transmission of B. burgdorferi from infected ticks usually requires a prolonged duration of attachment (>48 h), attached ticks should be removed promptly [ 5 , 6 ]. Persons should be taught to inspect themselves and their children's bodies and clothing daily after possible exposure to ixodid ticks. The body of the tick should be grasped with medium-tipped tweezers as close to the skin as possible and removed by gently pulling the tick straight out, without twisting motions. If some of the mouthparts remain embedded in the skin, they should be left alone, because they usually are extruded eventually; additional attempts to remove them often result in unnecessary damage to tissue and may increase the risk of local bacterial infection. Analysis of ticks to determine whether they are infected is not indicated, because the predictive values of such tests for the development of human disease are unknown.
Routine use of antimicrobial agents to prevent Lyme disease in persons who are bitten by a deer tick, even in areas of high endemicity, is not recommended because it is of unproven value and it is associated with potential risks as well as significant costs [ 44 , 55–58 ]. The vast majority of deer ticks (70%–80%), even in areas in which Lyme disease is highly endemic, are not infected with B. burgdorferi [ 58 ]. Moreover, the risk of infection after a recognized deer tick bite in an area of endemicity is estimated to be only ∼1.4% [ 57 ]. Three prospective controlled studies that compared antimicrobial prophylaxis with placebo to treat persons with tick bites were unable to demonstrate the effectiveness of antimicrobial prophylaxis in preventing Lyme disease because of the low risk of both infection and disease [ 57 ].
Serological testing for Lyme disease at the time of a recognized tick bite is not recommended [ 44 , 55 , 59 , 60 ]. Antibodies to B. burgdorferi present at the time that the tick is removed would probably be attributable to either a false-positive test result or to an earlier infection with B. burgdorferi , rather than to a new infection from the recent bite. Although some physicians obtain serum samples at the time of a tick bite and 6–8 weeks later to test for antibodies to provide reassurance, this practice usually is unnecessary, especially if it is likely that the tick was attached for <48 h. In addition, interpretation of serological tests in this setting is complicated by the problems described above (see Diagnosis).
Vaccines. Vaccines for Lyme disease that use recombinant OspA (rOspA) as the antigen have been produced by 2 manu-fac-turers and field-tested for safety and for efficacy in humans. LYMErix (SmithKline Beecham Pharmaceuticals, Philadelphia) contains 30 g of purified rOspA lipidated protein combined with 0.5 mg of aluminum adjuvant. This is the only licensed Lyme vaccine at this time. A vaccine produced by Pasteur-Mérieux-Connaught (Swiftwater, PA), Imulyme, contains 30 g of purified rOspA lipidated protein without adjuvant and is under review by the US Food and Drug Administration. Three doses of either vaccine are required for optimal protection; the second dose is given 1 month after the first dose and a third dose is given 12 months after the first dose. Preliminary data suggest that other immunization schedules (e.g., vaccination at 0, 1, and 6 months or at 0, 1, and 2 months) are safe and induce antibody responses similar to those induced by the immunization schedule of 0, 1, and 12 months [ 61 , 62 ]. However, at this time, only the schedule of 0, 1, and 12 months is approved by the US Food and Drug Administration.
This rOspA vaccine has a unique mode of action. OspA is expressed by B. burgdorferi that reside in the midguts of dormant ticks, but expression is later down-regulated in response to a blood meal. Because ticks must become engorged with blood before they can transmit the organism, patients with natural infection from B. burgdorferi have both little exposure and little antibody response to OspA (at least in the early stages of infection). When an immunized host is bitten by a tick infected with B. burgdorferi , protective OspA antibodies of the host are ingested by the tick. These antibodies then destroy the B. burgdorferi in the gut of the tick and thus prevent transmission to the host.
Two recent reports of large clinical trials in which either vaccine or placebo was administered at enrollment and again 1 and 12 months later have demonstrated the efficacy of the rOspA vaccine. In 1 trial, Steere et al. [ 63 ] found that the efficacy of LYMErix in preventing clinical Lyme disease was 49% (95% CI, 15%–69%) in the first year, after 2 injections. In the second year, after the third injection, the vaccine's efficacy in preventing symptomatic Lyme disease was 76% (95% CI, 58%–86%). Serological tests were done on subjects at entry and again 12 and 20 months later to detect asymptomatic infections with B. burgdorferi . The efficacy of this vaccine in preventing asymptomatic infection was 83% in the first year and 100% in the second year.
In another large clinical trial, Sigal et al. [ 64 ] found that the efficacy of Imulyme in preventing symptomatic Lyme disease was 68% (95% CI, 36%–85%) in the first year, after 2 injections [ 64 ]. In the second year, after the third injection, the vaccine's efficacy in preventing symptomatic Lyme disease was 92% (95% CI, 69%–97%). In this study, subjects with asymptomatic infections with B. burgdorferi were not identified. Some of the difference in the efficacy of the vaccines between the 2 studies may be due to differences in the methods of surveillance.
The rOspA vaccines used in both clinical studies appeared to be safe. The most frequently reported vaccine-related side effects were pain, redness, and swelling at the site of the injection. These effects were usually mild and self-limited. No hypersensitivity reactions were reported, and ≥30 days after the injections, there was no significant difference in either the type or the frequency of symptoms between recipients of vaccine and of placebo. There was also no significant difference in the frequencies of either severe or unexpected adverse effects between groups. In addition, there was no evidence that the vaccine exacerbated prior Lyme arthritis, caused neurological disease, or caused arthritis in subjects (including those with a history of Lyme disease).
The decision to recommend Lyme vaccine should be based on a determination of the person's risk of developing Lyme disease, which depends on the likelihood of being bitten by ticks infected with B. burgdorferi (for most people—even for those who live in areas of endemicity—the overall risk is relatively low). The rOspA vaccine does not protect all recipients from infection with B. burgdorferi and provides no protection against other tickborne diseases. Therefore, vaccinated persons should continue to practice personal protective measures against tick bites. Decisions regarding the use of rOspA vaccine should be based on an assessment of a person's risk of exposure to infected ticks and on a careful consideration of the relative risks and benefits of vaccination compared with other protective measures, including early diagnosis and treatment of Lyme disease. Specific recommendations have recently been published by the Public Health Service's Advisory Committee on Immunization Practices [ 65 ] and the Committee on Infectious Diseases of the American Academy of Pediatrics [ 66 ]. They can be summarized as follows.
Persons who reside, work, or recreate in areas of high or moderate risk. Lyme disease vaccination should be considered for persons aged 15–70 years who engage in activities that result in frequent or prolonged exposure to tick-infested habitats. Lyme disease vaccine can be considered for persons aged 15–70 years who are exposed to tick-infested habitats but whose exposure is neither frequent or prolonged. The benefit of vaccination beyond that provided by personal protective measures and early diagnosis and treatment of infection is uncertain. Lyme disease vaccine is not recommended for persons who have minimal or no exposure to tick-infested habitats.
Persons who reside, work, or recreate in areas of low or no risk. Lyme disease vaccine is not recommended for persons who reside, work, or recreate in areas of low or no risk.
Travelers to areas of high or moderate risk. Lyme disease vaccination should be considered for travelers to areas of high risk if frequent or prolonged exposure to tick-infested habitats is anticipated.
Data about antibody concentrations during a 20-month period after the first injection of rOspA vaccine indicate that subsequent booster doses may be necessary after the 12-month booster. However, additional data are needed before recommendations can be made about administration of >3 doses of rOspA vaccine.
Extensive (often inaccurate) publicity about both the risks and the outcomes of Lyme disease has produced considerable anxiety about this disease (many states with no endemic disease have Lyme disease support groups organized by patients). This concern has also led to inappropriate use of serological tests for Lyme disease as a screening test (often ordered as a result of requests by patients) in an attempt to identify the cause of widely prevalent, nonspecific symptoms such as pain and fatigue [ 40 , 67 , 68 ]. This has, in turn, led to a virtual epidemic of overdiagnosis and overtreatment of patients for Lyme disease, which only serves to perpetuate the myth that Lyme disease is commonly associated with severe, long-term morbidity [ 69–77 ]. Most studies indicate that with rare exceptions, the outcomes for persons with Lyme disease are excellent [ 12 , 13 , 78–82 ]. It is important for clinicians to consider what evidence (both clinical and epidemiological) there is to “rule in” Lyme disease before serological tests are ordered to rule out the diagnosis. Persons with only nonspecific symptoms and no objective signs of Lyme disease are very unlikely to have Lyme disease, regardless of the results of serological tests [ 40–42 ]. Inappropriate use of these tests frequently will result in misdiagnosis of Lyme disease and may prevent or delay the patient from receiving appropriate care for the true problem.
Google Scholar
Financial support: National Institutes of Health (RR-06022, AI-01703). E.D.S. has received research support and consulted for Pasteur Mérieux Connaught Laboratories and has given lectures that have been supported by Glaxo-Wellcome and by SmithKline Beecham, companies that manufacture products to prevent or treat Lyme disease.
- lyme disease
Email alerts
More on this topic, related articles in pubmed, citing articles via, looking for your next opportunity.
- X (formerly Twitter)
- Recommend to your Library
Affiliations
- Online ISSN 1537-6591
- Print ISSN 1058-4838
- Copyright © 2024 Infectious Diseases Society of America
- About Oxford Academic
- Publish journals with us
- University press partners
- What we publish
- New features
- Open access
- Institutional account management
- Rights and permissions
- Get help with access
- Accessibility
- Media enquiries
- Oxford University Press
- Oxford Languages
- University of Oxford
Oxford University Press is a department of the University of Oxford. It furthers the University's objective of excellence in research, scholarship, and education by publishing worldwide
- Copyright © 2024 Oxford University Press
- Cookie settings
- Cookie policy
- Privacy policy
- Legal notice
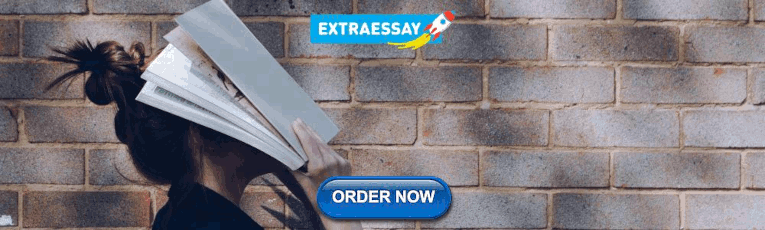
This Feature Is Available To Subscribers Only
Sign In or Create an Account
This PDF is available to Subscribers Only
For full access to this pdf, sign in to an existing account, or purchase an annual subscription.
- Skip to primary navigation
- Skip to main content
- Skip to primary sidebar
- Skip to footer
Johns Hopkins Medicine Lyme Disease Research Center
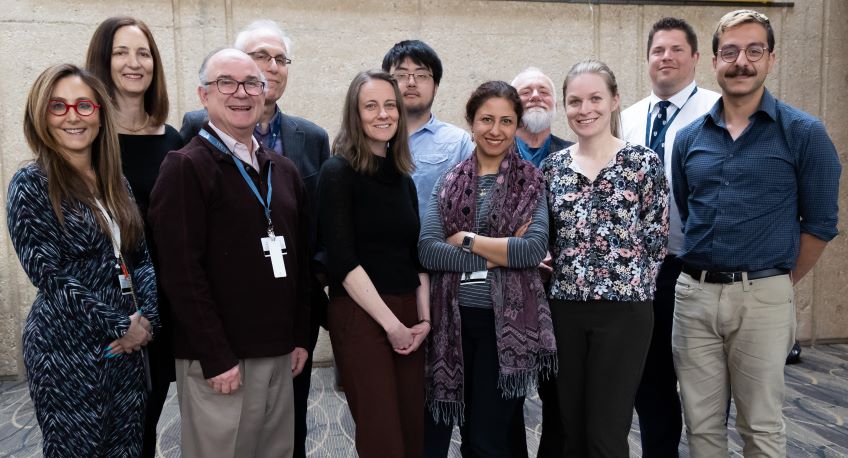
Patient-based Epidemiologic and Clinical Translational Research
The Johns Hopkins Medicine Lyme Disease Research Center is pioneering patient-based multidisciplinary research in all manifestations of Lyme disease.
Our mission is to bridge the gaps in Lyme disease knowledge and translate our research findings into improved patient care.
Disease Information
Lyme disease is a multi-system bacterial infection caused by the spirochete, Borrelia burgdorferi , which is transmitted to humans through the bite of an infected deer tick. In early Lyme disease patients can present with a rash and/or flu-like symptoms, but in later stages symptoms can manifest in multiple body systems including musculoskeletal, heart, brain, and nervous system.
Our multidisciplinary clinical and research team at the Johns Hopkins Medicine Lyme Disease Research Center conducts groundbreaking research to improve the understanding of Lyme disease and its varied manifestations. Our goal is to translate our pioneering research into improved patient care, education, and health outcomes.
Latest in Lyme Disease Research
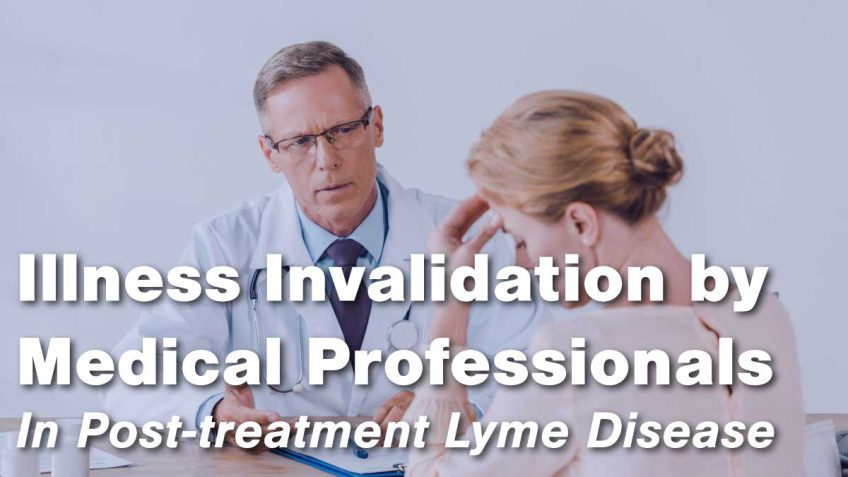
Invalidation by Medical Professionals in Post-Treatment Lyme Disease
Lyme Disease Research , Lyme Disease Research Highlights
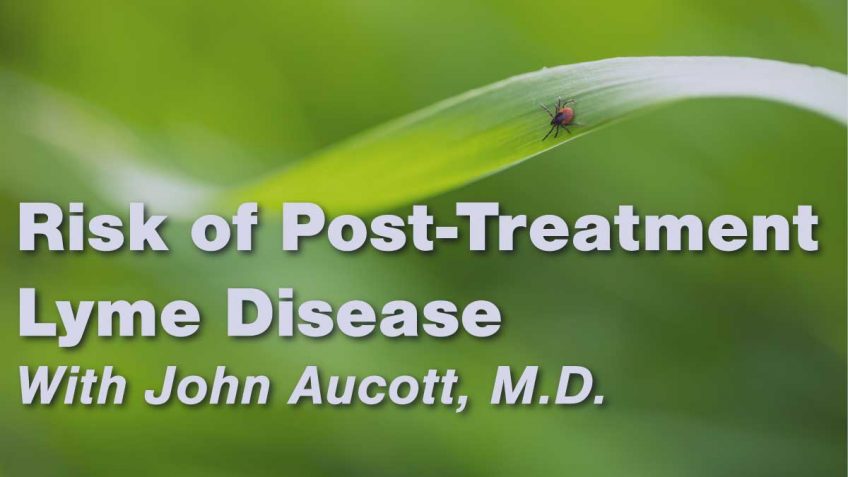
Risk of post-treatment Lyme disease in patients with early diagnosed and promptly treated Lyme disease: A prospective cohort study
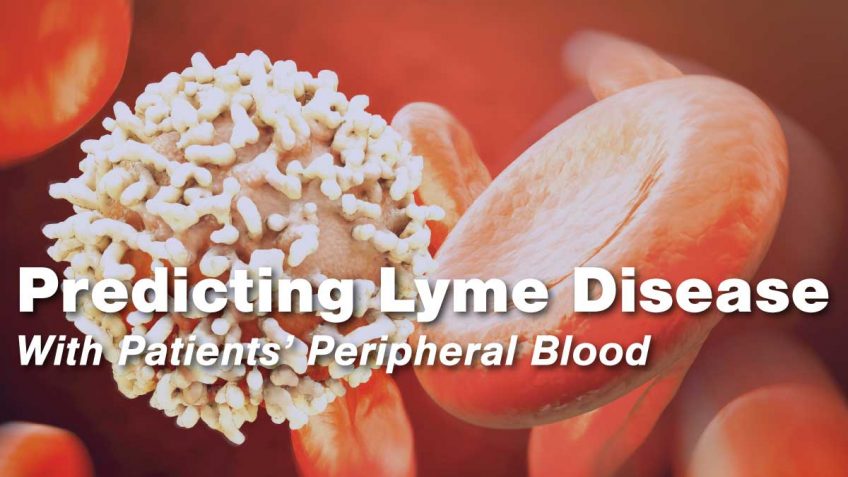
Predicting Lyme Disease from Patients’ Peripheral Blood Mononuclear Cells Profiled with RNA-Sequencing
Lyme disease resources.
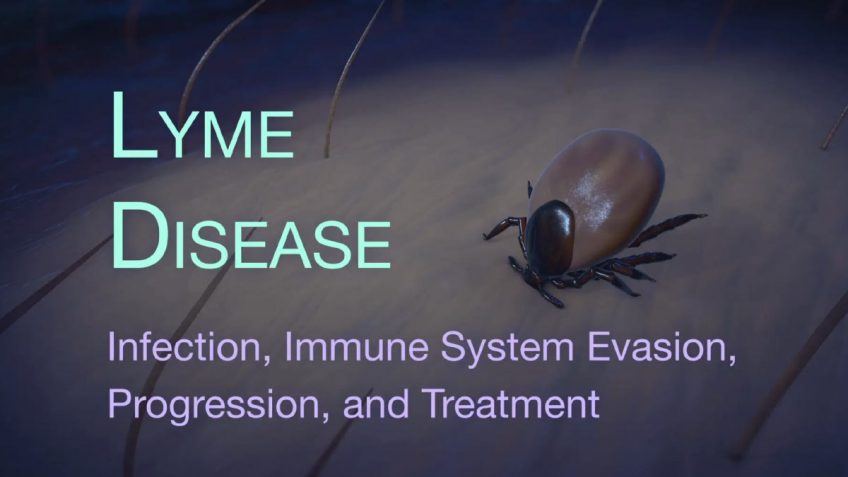
Lyme Disease Animation: Infection, Immune System Evasion, & Progression
Lyme Disease Video Education Resources
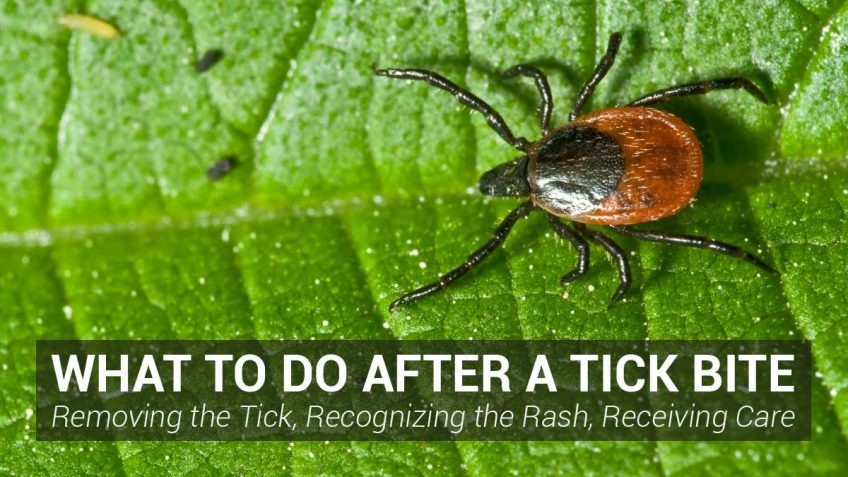
What to Do After a Tick Bite
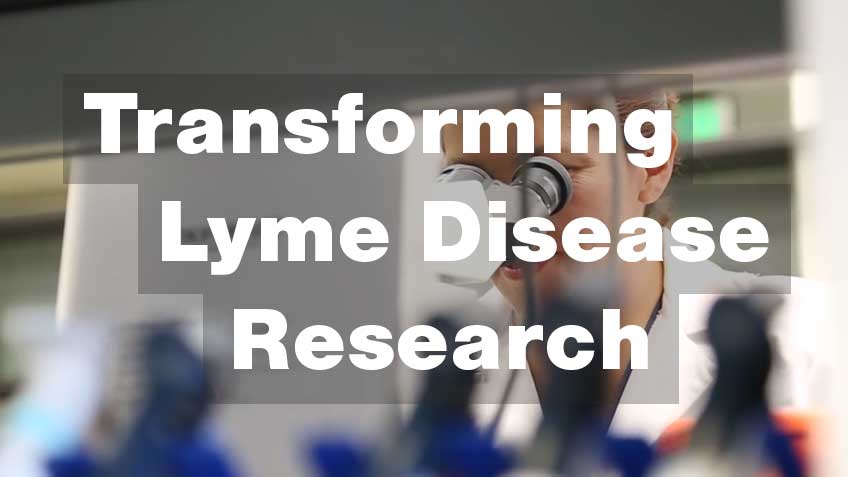
Transforming Lyme Disease Research
- Johns Hopkins Rheumatology
- Arthritis Center
- Lupus Center
- Myositis Center
- Scleroderma Center
- Sjögren’s Syndrome Center
- Vasculitis Center
Connect with Us
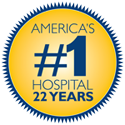
An official website of the United States government
Official websites use .gov A .gov website belongs to an official government organization in the United States.
Secure .gov websites use HTTPS A lock ( Lock Locked padlock icon ) or https:// means you've safely connected to the .gov website. Share sensitive information only on official, secure websites.
- Publications
- Account settings
- Advanced Search
- Journal List

The Accuracy of Diagnostic Tests for Lyme Disease in Humans, A Systematic Review and Meta-Analysis of North American Research
Lisa a waddell, mariola mascarenhas, shannon harding, robbin lindsay, nicholas ogden.
- Author information
- Article notes
- Copyright and License information
Competing Interests: The authors have declared that no competing interests exist.
- Conceptualization: LW JG MM SH RL NO.
- Data curation: LW.
- Formal analysis: LW.
- Investigation: LW JG SH MM.
- Methodology: LW JG SH MM.
- Project administration: LW JG.
- Resources: LW JG MM SH RL NO.
- Software: LW.
- Supervision: JG NO.
- Validation: LW JG MM SH RL NO.
- Visualization: LW.
- Writing – original draft: LW.
- Writing – review & editing: JG MM SH RL NO.
‡ These authors also contributed equally to this work.
* E-mail: [email protected]
Contributed equally.
Received 2016 Aug 25; Accepted 2016 Dec 2; Collection date 2016.
This is an open access article distributed under the terms of the Creative Commons Attribution License , which permits unrestricted use, distribution, and reproduction in any medium, provided the original author and source are credited.
There has been an increasing incidence of Lyme disease (LD) in Canada and the United States corresponding to the expanding range of the Ixodes tick vector and Lyme disease agent ( Borrelia burgdorferi sensu stricto) . There are many diagnostic tests for LD available in North America, all of which have some performance issues, and physicians are concerned about the appropriate use and interpretation of these tests. The objective of this systematic review is to summarize the North American evidence on the accuracy of diagnostic tests and test regimes at various stages of LD. Included in the review are 48 studies on diagnostic tests used in North America published since 1995. Thirteen studies examined a two-tier serological test protocol vs. clinical diagnosis, 24 studies examined single assays vs. clinical diagnosis, 9 studies examined single immunoblot vs. clinical diagnosis, 7 studies compared culture or PCR direct detection methods vs. clinical diagnosis, 22 studies compared two or more tests with each other and 8 studies compared a two-tiered serological test protocol to another test. Recent studies examining the sensitivity and specificity of various test protocols noted that the Immunetics® C6 B . burgdorferi ELISA™ and the two tier approach have superior specificity compared to proposed replacements, and the CDC recommended western blot algorithm has equivalent or superior specificity over other proposed test algorithms. There is a dramatic increase in test sensitivity with progression of B . burgdorferi infection from early to late LD. Direct detection methods, culture and PCR of tissue or blood samples were not as sensitive or timely compared to serological testing. It was also noted that there are a large number of both commercial (n = 42) and in-house developed tests used by private laboratories which have not been evaluated in the primary literature.
Introduction
Lyme disease (LD) is the most common tick-borne infection in North America [ 1 , 2 ]. It was first publically recognized in the United States in 1975 in the towns of Lyme and Old Lyme Connecticut as a result of an investigation into 51 cases (39 children) with a similar form of arthritis, although the first case was describe five years earlier by a dermatologist in Wisconsin [ 3 , 4 ]. In North America early signs of infection may include erythema migrans (EM, a characteristic skin rash that often has a bulls eye appearance) and fever and non-specific symptoms like headache and lethargy [ 5 , 6 ]. If untreated, the disease can progress to disseminated LD with neurological, cardiac and arthritic manifestations [ 7 ]. Lyme disease in North America is caused by Borrelia burgdorferi sensu stricto (hereafter called B . burgdorferi ) and recently Borrelia mayonii was identified and may be responsible for a proportion of cases, however the performance of LD diagnostic tests to identify B . mayonii infection is not available [ 8 ]. In Europe B . afzelii , B . garinii , B . burgdorferi , B . spielmanii , B . bissettii and B . bavariensis cause disease with a wider variety of symptoms than reported in North America; a number of genospecies including B garinii occur in Asia.
Ticks of the genus Ixodes transmit the spirochete when they feed. Ixodes scapularis , the blacklegged tick, is the main vector in northeastern and upper midwestern United States and Canada while I . pacificus is the major vector in western United States and western Canada [ 9 , 10 ]. The primary vectors of LD in Europe and Asia are I . ricinus and I . persulcatus respectively [ 6 , 11 ]. The principal natural hosts of immature stages of the ticks and B . burgdorferi include rodents, other small and medium sized mammals, reptiles and birds, while adult female ticks feed mainly on deer [ 12 ].
Lyme disease incidence has increased since 1975 as the tick vectors have expanded their geographic range across the north eastern and upper mid-western states in the US and more recently into Canada [ 2 , 13 ]. Range and spread of ticks and B . burgdorferi is facilitated by migratory birds and terrestrial hosts [ 14 ]. There is increasing evidence that climate change will result in further northward expansion of the tick vector’s range in Canada, resulting in increased future risk of LD among Canadians [ 15 , 16 ].
The diagnostic tests available for confirmation of human LD have variable sensitivity and specificity depending on the stage of infection, thus it is important to monitor the literature on available tests for LD to promote those tests that perform the most effectively and address concerns about the performance of non-validated tests and test protocols using evidence-informed strategies for decision making [ 17 , 18 ]. Currently in Canada and the United States, a two-tiered serology protocol is the only validated diagnostic approach for LD diagnosis recommended by United States CDC and the Public Health Agency of Canada [ 17 , 18 ]. This two-tiered test is typically an enzyme immunoassay (EIA) to detect IgM or IgG antibodies to B . burgdoferi in serum and if the sample is positive or equivocal on the screening assay, then a western blot is used to detect serum IgM or IgG antibodies to B . burgdorferi . Use of IgM testing is recommended during the first 30 days of infection, after which only IgG tests should be used. Currently, only serology tests have been licensed for use by the FDA and the Health Canada Medical Devices Branch (HC) for LD testing [ 19 , 20 ]. Other direct detection tests such as PCR may be commercially available, but they have not been licensed for use by a governing body. There are a number of commercial EIA kits that are licensed by the FDA and/or HC and use either whole cell preparation of B . burgdorferi and/or purified recombinant or chimeric antigens (see S2 Text ). Other EIAs reported in the literature have been developed within the reporting laboratory and have not been commercialized or under-gone licensing and will be referred to as in-house developed tests [ 21 , 22 ]. The EIA’s have good sensitivity after 30 days of infection, but typically suffer from lower specificity [ 22 ]. In 1995, the Centers for Disease Control and Prevention (CDC) adopted criteria for interpreting the results of the western blot for LD and most commercialized tests follow these guidelines [ 23 ].
The objective of this systematic review is to summarize the North American evidence on the accuracy of diagnostic tests and test regimes used to diagnose LD in patients presenting with clinical symptoms in North America at various stages of disease and to address the question of whether there is evidence of superior, equivalent or poor performance by the commercial (approved by the FDA and/or HC) and in house laboratory tests captured in this review. To the best of our knowledge this systematic review is a significant update to Dumler (2001) [ 24 ] and is complementary to a recent systematic review on European Lyme disease diagnostic tests [ 25 ].
Scoping review
This systematic review was preceded by a scoping review conducted by Greig et al (2016) to identify, classify and characterise what is the current state of scientific knowledge on surveillance methods, prevention and control strategies, diagnostic tests, risk factors, and societal attitudes and perceptions towards LD in humans and B . burgdorferi in tick vectors and vertebrate reservoirs [ 26 ]. Briefly, the scoping review methodology was designed to characterise the primary literature on LD in humans or B . burgdorferi tick vectors or reservoirs, thus studies not on LD or B . burgdorferi were excluded from the scoping review. Additionally, the primary research had to address one of the following topics: surveillance/monitoring, prevalence, incidence, societal attitudes and perceptions in North America and global prevention and control strategies, diagnosis and risk factors. Research on clinical LD and treatment were considered outside the scope of this review. Each relevant paper was classified by purpose, study design, location of the study, B . burgdorferi , host species investigated, vector species investigated, sampling dates, diagnostic tests used, and whether the paper contained extractable data.
The scoping review search strategy was developed and pretested by three individuals with extensive experience in knowledge synthesis, zoonotic diseases and library science. The following search algorithm was implemented in eight bibliographic databases: BIOSIS (via web of knowledge), CAB abstracts, Scopus, PubMed, PsycINFO, APA PsycNet, Sociological Abstracts, and EconLit with no limitation on the search, this was followed by a comprehensive search for grey literature [ 26 ]: (lyme OR borrelia) AND ("host" OR sentinel OR landscaping OR "vector" OR "vectors" OR "monitor" OR "monitoring" OR surveillance OR reservoir OR reservoirs OR prevalence OR educate OR education OR barrier OR barriers OR intervene OR intervention OR incidence OR rate OR prevent OR prevention OR control OR risk OR risks OR attitude OR attitudes OR perception OR perceptions OR diagnostic). The search was conducted September 13 th -14 th , 2013 and no update of the search has been performed as analysis indicated the findings would not change with the addition of new papers, thus the resources required to conduct the update were not prioritized. The protocol for the scoping review is available upon request.
Systematic review methods
Studies identified in the scoping review that evaluated diagnostic tests for humans were fully evaluated in this systematic review. The systematic review tools include a confirmation of relevance, location of study, availability of extractable data and a quality assessment form based on the Quality Assessment of Diagnostic Accuracy Studies (QUADAS-2) tool [ 27 – 29 ]. This tool assesses the risk of bias and other methodological quality domains to evaluate the extent to which the results of each study or group of studies could be biased. The QUADAS-2 tool assessed the four quality domains ( Table 1 ) with respect to patient selection, the diagnostic tests used, the reference standard and flow and timing of the study [ 28 ]. An additional section was added to evaluate comparison tests and capture the presence of funding bias [ 30 , 31 ].
Table 1. Number of studies meeting each quality criteria in QUADAS-2 based on 48 articles from the United States examining the accuracy of diagnostic tests for Lyme disease included in this systematic review.
The data extraction form captured all pertinent study details and results. The systematic review was managed in DistillerSR (Evidence Partners, Ottawa, ON, Canada) a web-based systematic review management software. Each form was completed by two reviewers working independently and conflicts were resolved by consensus. Data were exported to Microsoft Excel 2010 (Microsoft Corp., USA), prepared for summarization and analysed in STATA v. 13 (StataCorp., USA). The study protocol and PRISMA evaluation can be found in the supplementary material ( S1 Text , S3 Text ).
Included papers examined the accuracy of diagnostic tests for LD in North America after 1995, and included studies that compared results of one test using a validated test panel, results of clinical diagnosis, or a gold standard test result or investigated inter-test agreement. The recommendations for two-tier testing occurred in 1995, so we limited the review to studies conducted after 1994. Studies that screened an asymptomatic population for LD were excluded from this study. No inclusion or exclusion criteria were implemented on the type of control group; instead it was evaluated as a source of variation between study results (heterogeneity). The control group was usually a mix of one or more categories of healthy volunteers from non-LD endemic or LD endemic regions, or asymptomatic blood donors. In some studies, patients with diseases that have similar signs and symptoms to LD or have humoral responses that overlap with LD and are known to cross-react (e.g. rheumatoid arthritis, systemic lupus erythematosus, syphilis, autoimmune disorders, leptospirosis, periodontitis, relapsing fever, tularemia, Southern Tick-associated Rash Illness (STARI), multiple sclerosis, and Epstein-Barr virus infection) were included as controls to more precisely define test specificity. Studies often used well-defined samples from serum repositories or panels, like those developed by CDC [ 32 ], a research institute [ 33 , 34 ] or a commercial company [ 35 ]. These results were included in this systematic review and the impact of patient-based or panel samples on the outcome was investigated.
For this review the stages of LD are as follows: Early / acute LD (stage 1) is defined as those patients presenting with EM and/or associated manifestations that have experienced signs and symptoms of LD for less than 30 days [ 7 ]. Stage 2 illness is early disseminated LD, which includes manifestations of early neurological LD, cardiac LD and multiple EMs [ 36 ]. Stage 3 is late LD, typically with manifestations of Lyme arthritis and late neurological LD [ 36 ]. Those patients tested after antibiotic therapy are described as convalescent with the stage of LD assigned prior to treatment. Post treatment Lyme syndrome is defined as a condition where despite treatment the patient continues to experience illness [ 37 ]. “Chronic LD” is a condition that is not recognised as being caused by B . burgdorferi by most infectious disease experts, occurs in patients exhibiting non-specific illness who do not test positive on Food and Drug Administration (FDA) approved serological tests, so these have been excluded from this review [ 38 ].
Meta-Analytic Methods
The dataset was managed in MS excel; each line of data represents a single test accuracy outcome and one study may have several comparisons, thus several lines of data. Each comparison was extracted, grouped and coded according to tests and type of outcome reported. When there were four or more lines of data for a category, meta-analysis was conducted using hierarchical logistic regression and bivariate models in Stata 13 using Metandi and Midas command packages. These models have been designed to account for the correlation between sensitivity and specificity [ 39 ] and they overcome the often violated assumptions of a linear regression model [ 40 , 41 ]. These hierarchical models use 2x2 cell counts to compute log transformations of proportions for the analysis [ 39 ]. Without covariates, the hierarchical summary receiver operating characteristic (HSROC) and bivariate models are equivalent although their assumptions are different: HSROC assumes there is an underlying Receiver-Operating Characteristic (ROC) for each study and the bivariate model directly models the log-odds transformed sensitivity and specificity assuming a bivariate normal distribution between studies [ 42 ].
Meta-analytic statistical summaries of sensitivity, specificity, likelihood ratios and diagnostic odds ratio have been summarized where possible in the tables. Model diagnostics including goodness of fit, normality, influential and outlying points, publication bias and heterogeneity were examined where possible. Publication bias was not evaluated when heterogeneity was >60% or there were less than 10 lines of data. Meta-regression using the bivariate model was used to examine whether predetermined covariates explain some of the between-study variation given there was sufficient data to fit the model (>10 data lines per covariate).
In the scoping review, 485 articles focused on diagnosis of LD in humans globally and were further evaluated for inclusion in this systematic-review meta-analysis. The decision tree for selection of articles and reasons for exclusion of potentially relevant studies in this systematic review is shown in Fig 1 . Forty-eight relevant diagnostic test evaluations conducted in North America between 1995 and 2013 were included in this systematic review (see S2 Text and S1 Dataset ).
Fig 1. Flow diagram of diagnostic test papers through the systematic review from the scoping review.
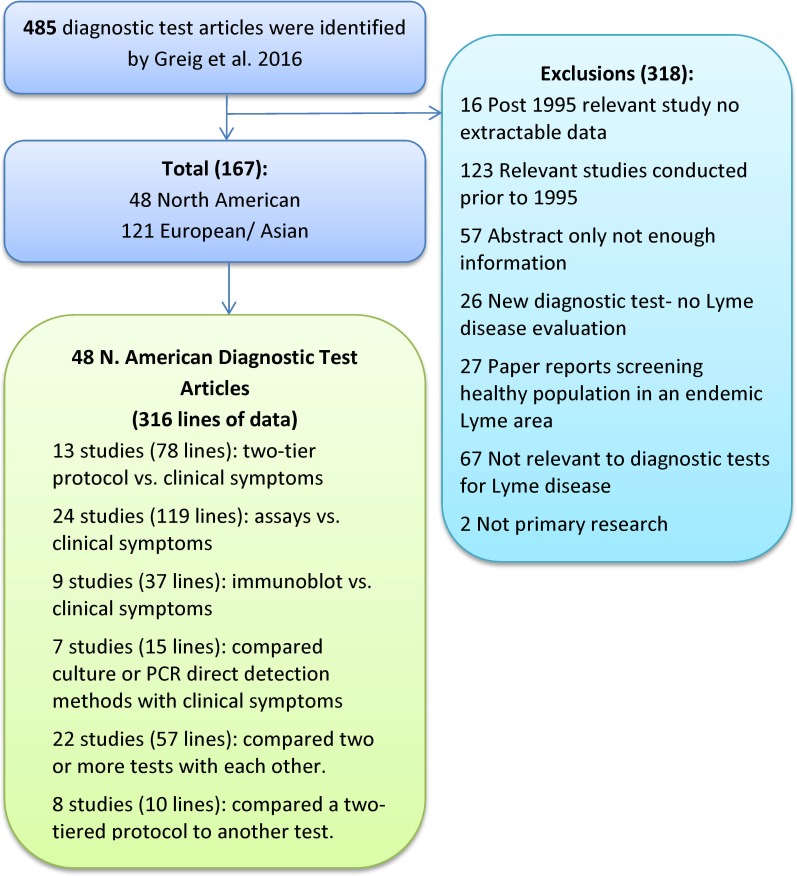
The QUADAS-2 tool results, Table 1 , indicated that there was an unclear risk of bias in 84% of studies, meaning the study received an unclear or high risk of bias score on one or more domains (see S1 Dataset ). No studies were excluded from the analysis based on their QUADAS assessment. In two studies it was apparent that the sample population was not appropriately enrolled in the study as the case population and control population were enrolled at different times and places, which could lead to biased (exaggerated) results for test accuracy [ 43 , 44 ]. Appropriate blinding was often not addressed in many papers and unexplained exclusion of observations from the analysis was another common reporting issue. Many of the studies (28.6%) had authors employed by or funded by commercial companies that supplied one or more of the tests evaluated. In four of these studies the risk of funding bias was identified to be very high [ 43 , 45 – 47 ].
The diagnostic accuracy of tests compared to clinical diagnosis
Two-tier serological test vs. clinical diagnosis.
Thirteen studies evaluated the two-tier serological test protocol for diagnosis of LD at different stages of disease and after antibiotic therapy. Table 2 provides the meta-analytic summaries demonstrating low sensitivity, 46.3% (95%CI 39.1–53.7), for early (stage 1) LD patients and increasing sensitivity with stage 2, 89.7% (78.3–95.4), and stage 3, 99.4% H (95.7–99.9) LD. There was relatively high specificity (98.3%–99.9%) across control groups. Most false positives within the control groups were patients with diseases known to produce antibodies that cross-react in serological tests for B . burgdorferi . Nine studies (14 lines of data) presented results for two-tier serological testing where at least one of the tests was not FDA licensed (designed in house by the reporting laboratory), Table 2 . Heterogeneity analysis of sensitivity and specificity on the impact of using non-commercial tests was not significant. At the early stage of LD the two-tier testing method was good for ruling in LD if the patient tested positive, but had very poor predictive value for ruling out LD, which is why it is recommended to retest after 30 days [ 21 ]. However, for convalescent patients treated at stage 1 LD sensitivity remained low even after 30 days.
Table 2. Thirteen studies (78 lines of data) evaluating a two-tier serological test protocol summarized by the stage of Lyme disease tested using a hierarchical logistic regression model in Stata 13 or the range of sensitivity and specificity data presented in the paper when less than four lines of data were available for meta-analysis.
Sn estimate/ Sp estimate are from the meta-analysis bivariate model unless otherwise noted.
* Summary sensitivity and specificity across all tests at the specified stage of LD.
ǂ Value or range of values for sensitivity and specificity as reported by the author.
Sn = sensitivity, Sp = specificity, DOR = diagnostic odds ratio. LR+ (positive likelihood ratio) and LR- (negative likelihood ratio) are based on the bivariate model and are different than direct calculations of LR+/LR- [ 48 ]. ELISA = enzyme-linked immunosorbent assay
H Based on I 2 , a measure of between study heterogeneity, the heterogeneity in this group of studies was <60%, thus considered to be homogenous.
FDA = Food and Drug Administration approved, HC = Health Canada approved, NC = non-commercial
Vidas = Vidas Lyme Screen, Wampole = Wampole Bb (IgG/IgM) ELISA test system, Marblot = MarDx Lyme Disease (IgG and IgM) Marblot Strip Test System, Virablot = ViraMed Biotech Borrelia B31 (IgG or IgM) Virablot, Immunetics C6 = Immunetics® C6 B . burgdorferi ELISA™, Cambridge = Cambridge, Human Lyme EIA for detection of antibodies, IB = immunoblot, Zeus ELISA = Zeus Lyme IgG or IgM ELISA Test system, Zeus AtheNa = Zeus AtheNA Muti-Lyte test system, Liason = Liason Borrelia IgG /IgM assay model 310870 (CLIA)
One study (1 line of data) was excluded from the analyses ([ 34 ]) because there was no specificity reported in the paper.
EIA vs. clinical diagnosis
First tier serological tests including enzyme-linked immunosorbent assays (ELISA) and other serological assays were evaluated in 23 studies (119 lines of data) with well-defined and whole cell targets, Table 3 . There were a mix of FDA-licensed tests and in house tests. Similar to the two-tiered tests, test performance for patients with stage 1 LD was highly variable and had poor sensitivity. In later stages of LD, the sensitivity improved. The overall specificity varied by test and between studies more than was reported for the two-tier tests.
Table 3. Twenty three studies (119 lines of data) evaluating different assays (mainly 1 st tier tests) by stage of Lyme disease using hierarchical logistic regression models or simply sensitivity and specificity when less than four lines of data were available for meta-analysis.
Sn estimate/ Sp estimate are from the meta-analysis bivariate model.
* Summary sensitivity and specificity across all test at the specified stage of LD.
¥ There was a significant difference between the commercial and in house test results.
IHA = indirect hemagglutination antibody test, ELISA = enzyme-linked immunosorbent assay, LIPS = luciferase immunoprecipitation systems, IFN-y = Interferon gamma, fla = flagellum, Osp = Outer surface protein.
ELISA performance on early stage 1 LD was investigated in 53 lines of data (16 studies), Table 3 . These were further grouped by type of ELISA to understand where variation between studies was occurring. ELISAs targeting C6 included 4 lines (3 studies) on the Immunetics® C6 B . burgdorferi ELISA™ kit and seven lines (four studies) on unlicensed C6 ELISAs ( Table 3 ). Accounting for whether the C6 ELISA was licensed explained 27% of the heterogeneity between studies and indicated the commercial ELISAs had an insignificant higher sensitivity 91(81–100) vs. 64(47–80) and similar specificity 97(94–100) vs. 97(95–99) over all stages of LD.
Whole cell sonicate (WCS) ELISAs for early LD included 10 lines from 6 studies Table 3 . Three commercial test kits were included; Lyme Stat Test Kit, VIDAS Lyme Screen II and Wampole Bb ELISA test system (see S2 Text ) across six lines and three studies. These performed differently than the four in house WCS ELISAs and the authors did not offer an explanation for the divergent results.
Recombinant proteins and/or chimeric proteins from Osp A-F (mainly A and C) targets were used to develop assays and tested on early LD patients. All studies were based on in house ELISAs with small sample sizes and the reported sensitivities varied from target to target ranging from 0–86%. Other assays included the use of Poly-ethylene Glycol (PEG)-peptide conjugates in an ELISA that reported 100% sensitivity and specificity on a small sample [ 49 ]. An indirect hemagglutination antibody (IHA) test using B . burgdorferi strains B31 and B126 had a low sensitivity 46–48% and a specificity of 98–99% which is comparable to other tests for early LD [ 50 ].
For assays used in cases of late LD, the sensitivity and specificity were higher and more consistent compared early LD results. A meta-regression controlling for test in the late LD category showed the Immunetics® C6 B . burgdorferi ELISA™ significantly out-performed (sensitivity and specificity p≤0.001) in house C6 ELISAs, the commercial WCS ELISAs (VIDAS Lyme Screen II and Wampole Bb (IgG/IgM) ELISA test system) and the in house ELISAs using various recombinant/ chimeric Osp targets, Table 3 .
Not included in the meta-analyses in Table 3 was an evaluation of the reactivity of individuals previously vaccinated with the Osp A vaccine (removed from use in 2002); the results showed a 95% false positive rate with a WCS ELISA and a 5% false positive rate with a recombinant Osp A ELISA [ 51 ].
Immunoblots vs. clinical diagnosis
Across nine studies several commercial western blots were evaluated against clinical diagnosis of a range of LD. These included the Marblot test strip system by MarDx®, the Boston Biomedica Inc.(BBI) B . burgdorferi western blot test kit®, Immuno Dot Borrelia Dot Blot Test® and Viramed Biotech B . burgdorferi B31 Virablot®. Only one in house immunoblot was evaluated investigating the diagnostic sensitivity of a few recombinant targets.
The MarDx ® Lyme Disease Marblot Strip test system was evaluated in four studies (7 lines of data) on select LD groups and across early to late LD groups [ 52 – 55 ]. A meta-regression controlling for group indicated that the test performed significantly better on late LD patients, but whether the investigator evaluated results for IgM, IgG or both in parallel did not significantly affect the sensitivity or specificity.
The BBI western blot was evaluated in two separate studies using the same CDC test panel, but slightly different classification criteria; one used the BBI criteria (IgG required 3+ bands of 20,23,31,34,35,39 and 83 kDa and IgM 2+ bands 23,39,41, and 83 kDa) which has a different formulation for positive samples compared to the CDC criteria (IgG required 5+ bands 18, 23, 28, 30, 39, 41, 45, 58, 66, and 83 to 93 kDa and IgM 2+ bands 23, 39, and 41kDa) [ 35 , 46 ]. The results of the two criteria differed in sensitivity, which was 77% and 93% using CDC criteria respectively for IgM and IgG blots, compared to 93% and 100% using the BBI criteria for IgM and IgG, however the difference was not significant and specificity ranged from 77–99% with a gain in sensitivity resulting in slight losses to specificity, Table 4 .
Table 4. Eight studies (33 lines of data) evaluating different immunoblots (2nd tier tests) at all stages of Lyme disease using hierarchical logistic regression models or the sensitivity and specificity data when less than four lines of data were available for meta-analysis.
* IgM and IgG western blot tests conducted on early Lyme disease patients (<30 days) and only IgG tests conducted on later stages (>30 days) as per CDC guidelines.
s subjective test interpretation: technician assesses banding pattern and line intensity. po partially objective test interpretation: technician assesses dot intensity.
ǂ value or range of values for sensitivity and specificity as reported by the author.
Sn = sensitivity, Sp = specificity, DOR = diagnostic odds ratio. LR+ (positive likelihood ratio) and LR- (negative likelihood ratio) are based on the bivariate model and are different than direct calculations of LR+/LR- [ 48 ].
The Immunodot Borrelia Dot Blot IgG/IgM test by General Biometric Inc. was examined in one study; the results are shown in Table 4 . An insignificant increasing trend in sensitivity with disease progression was noted (stage 1 50% (95%CI 19, 87), stage 2 70% (35, 93) and stage 3 100% (63, 100) [ 52 ]. Viramed Biotech Borrellia burgdorferi B31 IgG/IgM Virablot demonstrated a comparable sensitivity and specificity in one small study to the other immunoblots evaluated [ 35 ]. One in house recombinant immunoblot (data not shown) did not perform well in the published study with sensitivities ranging from 7 to 60 percent for different targets [ 56 ].
Tests for direct detection of Borrelia burgdorferi by bacterial isolation or PCR vs. clinical diagnosis
There are six studies, (13 lines of data) that examined bacterial isolation by culture and PCR detection of B . burgdorferi in a variety of human samples from cases of early and disseminated LD [ 57 – 62 ]. Meta-analysis was not possible within this group of studies because there were not enough lines of data within each detection method. The most commonly used medium is Barbour-Stoener-Kelly (BSK) medium, which has been modified by some authors to improve its sensitivity [ 63 ]. Three studies attempted to isolate B . burgdorferi from blood (serum/plasma) of patients with early LD (stage 1) and the sensitivity of this approach was 27%, 71% and 94% [ 57 – 59 ]. With respect to the latter sensitivity, it has been suggested that laboratory contamination may account for the very high sensitivity reported [ 64 ]. Two studies reported sensitivities of 62–81% from biopsy samples of EM during early LD [ 59 , 60 ], although both sample sizes were very small. Phillips et al. evaluated an “MPM” medium for detection of B . burgdorferi in the blood of LD patients that had been previously treated, but then relapsed [ 65 ]. They reported a sensitivity of 91.5% in these patients, however two studies were unable to reproduce these results and both demonstrated that the BSK-H culture was superior [ 66 , 67 ].
Three studies (eight lines of data) were captured with information on the use of PCR to identify B . burgdorferi in early LD [ 59 , 61 , 62 ]. Samples included blood and tissue biopsies and each PCR targeted different primers. Eshoo et al (2012) used blood samples and multi-loci PCR targeting eight different loci to both detect and genotype B . burgdorferi , the sensitivity was 62% (40–79) and the specificity was 100% [ 61 ]. Liveris et al (2012) used a nested PCR on serum samples and biopsy samples with a sensitivity of 40.6 and 42.6% respectively [ 59 ]. They also implemented a qPCR on plasma samples demonstrating a sensitivity of 33.8%. Two nested PCR primer sets targeting the Osp A gene were investigated in neurological LD, both acute and late cases using cerebral spinal fluid samples; they reported a sensitivity of 37.5–50% in acute cases and 12.5–25% in late cases [ 62 ]. Across the direct detection studies sensitivity was low and in most cases lower than the two-tier test regime, assays or immunoblots reported for early LD.
Inter-test comparisons
The results of inter-test comparisons are summarised in Tables 5 – 7 . Note that in these tables we have positive agreement and negative agreement that indicate how well the two tests agreed to classify samples as positive or negative respectively. Thus, positive agreement is the probability that test 2 is positive if test 1 is positive and negative agreement is the probability that test 2 is negative if test 1 is negative. Table 5 has comparisons between the two-tier serological tests compared to other tests and Table 6 includes studies that examined various assays and immunoblots for agreement.
Table 5. Summary of agreement between tests reported in eight studies (10 lines of data) that examined two-tier serology testing compared to a different test or two-tier protocol.
a PA = positive agreement estimate = On a sample of clinical LD patients, this is the probability of test 2 being positive if test 1 is positive.
b NA = negative agreement estimate = On a sample of clinical LD patients, this is the probability of test 2 being negative if test 1 is negative.
NC = Not a commercial test, n/a = not applicable, E = early Lyme only (stage 1)
BSK = Barbour-Stoener-Kelly (BSK) medium, nPCR = nested polymerase chain reaction, qPCR = quantitative polymerase chain reaction
Table 7. Summary of agreement reported in four studies (six lines of data) evaluating culture and/or PCR of biopsy and various blood samples for the identification of Borrelia spp. in early stage1 Lyme disease patients.
E = early Lyme only (stage 1)
Table 6. Summary or range of agreement reported in 14 studies (51 lines of data) evaluating different tests paired against each other and tested on samples meeting the clinical definition of Lyme disease or a test panel.
a PA = positive agreement estimate = On a sample of clinical Lyme disease patients, this is the probability of test 2 being positive if test 1 is positive.
FDA = Food and Drug Administration approved, HC = Health Canada approved, n/a = not applicable
NC = Not a commercial test, P = test panel used, E = early Lyme only (stage 1)
EMIBA = Enzyme-linked capture immune complex biotinylated-antigen assay
Table 7 contains studies that looked at various samples and culture sensitivity in early LD as well as the use of various PCRs to identify B . burgdorferi infection. In one study there was agreement between culture of serum vs. plasma, however whole blood classified more samples positive compared to serum resulting in little agreement [ 58 , 68 ]. The confirmation of B . burgdorferi presence in culture using qPCR both increased the sensitivity and shortened the length of culture time before a positive result could be obtained [ 69 ]. A study examining the sensitivity of direct qPCR targeting flaB or recA genes compared to culture of 2mm EM biopsy samples showed little agreement and qPCR targeting the recA gene was more sensitive compared to the fla B target [ 70 ].
Diagnostic Test Performance in Early Lyme disease
Testing for LD in patients exhibiting signs and symptoms of LD for less than 30 days is challenging as the performance of available test protocols is not optimal for making clinical decisions. This is largely due to the time required for the infected individual’s immune system to mount a reaction. This is why researchers have explored the use of a variety of targets including VlsE and C6 expressed after infection, Osp C and Fla B expressed by the feeding tick to detect infection sooner [ 71 , 72 ]. However, cross-reactivity and genetic variability within the targets has limited the diagnostic performance of any single target [ 73 , 74 ]. Thus the results of expected sensitivities and specificities in Table 8 emphasize the importance of physician evaluation and informed judgement when deciding to treat rather than rely entirely on imperfect serological test protocols. Notable findings in the table include the higher specificity associated with the two-tier testing method and the poor and highly variable sensitivity of serological tests in the initial stages of disease when an individual is mounting an immune response to B . burgdorferi .
Table 8. Summary of the sensitivity and specificity of different testing options for early Lyme disease (stage 1) patients.
* Summary sensitivity and specificity across all tests on early LD.
IHA = indirect hemagglutination antibody test, Osp = Outer surface protein
The 48 studies included in this analysis were all conducted in the United States from 1995 onwards. The samples included patients or historical samples where the clinical presentation fit the diagnosis of LD. Within the results we summarized results for all stages of LD, separate stages 1–3 LD and convalescent stages 1–3 LD to facilitate an evaluation of trends, similarities and differences by test, stage of disease and treatment status. There were a few studies that differentiated acute samples <7 days and early Lyme samples 7–30 days, but not enough to analyse predictive values within early LD. Similarly there were studies that used culture positive patients exclusively, however the culture status of the patients did not significantly account for the heterogeneity. Stage 1, 2, and 3 convalescent LD groups were sampled in a number of studies and are summarized separately from samples drawn pre-treatment as it is known that there are differences in the immune response depending on the length of LD prior to treatment [ 5 , 6 ].
In the United States it was recently estimated that less than 12% of Lyme disease tests were for true infections [ 75 ]. The LD test results for patients who do not meet the clinical criteria can be used to rule out LD, but a positive test is likely to be a false positive. Thus, the over use of these assays to diagnose LD has been an on-going discussion and challenge for topic-specialists and physicians [ 76 ]. The literature summarised in this systematic review was based on research conducted from 1995 when the CDC adopted the recommendations for two-tier testing of LD acquired in North America. Their goal was to improve the specificity of LD testing by recommending the use of a sensitive EIA followed by a more specific western blot for positive and equivocal samples [ 23 ]. Most of the research on diagnostic tests in North America were based on serology, mainly antibody based assays detecting an immune response against B . burgdorferi . As of May 2015 there were 42 tests approved by the FDA for use in the United States and 22 approved by Health Canada Medical Devices Branch for use in Canada, however only a few of these tests were evaluated in the primary literature and all the literature published since 1995 was conducted in the United States (see S2 Text ).
Recent studies examining inter-laboratory agreement and the sensitivity and specificity of various test protocols noted that the C6 ELISA alone and the two-tier approach has superior specificity compared to proposed replacements and the CDC-recommended western blot algorithm has equivalent or superior specificity over other proposed test algorithms [ 77 ]. The findings of this review are in agreement with other authors that sensitivity was highest for ELISAs targeting C6 and these showed less variability in test sensitivity compared to other tests and test protocols [ 77 ]. The C6 ELISAs, particularly the commercial assays, had promising sensitivity, specificity and agreement of results with two-tier protocols, which is likely why the Immunetics® C6 B . burgdorferi ELISA™ has become widely used in place of some WCS assays. Although we did not summarize results of inter-laboratory agreement studies in this systematic review, the requirement for technical expertise and subjectivity in result interpretation for many LD tests, particularly western blots, contributes to poor agreement between technicians, tests and/or laboratories [ 77 ].
Factors that affect the sensitivity and interpretation of the results include type of sample and stage of disease in addition to possible variations in the type, target and conduct of the diagnostic tests. In this systematic review all relevant studies examining the efficacy of serological tests used serum samples from patients. There were no studies that employed the use of synovial fluid or cerebrospinal fluid for diagnosis of LD with serological assays. However in the last few years a number of studies have emerged from Europe on assays designed for cerebrospinal fluid samples in the diagnosis of neuroborreliosis which is a more common clinical presentation in Europe [ 78 – 80 ].
Throughout our results there was a positive association between duration of infection/ stage of disease and sensitivity of serological LD tests [ 34 , 47 , 60 ]. Thus, recommendations include re-testing after 30 days if the initial serological test was done during the early (non-disseminated) stages of infection and employing IgM assays as well as IgG assays to detect early immune reactions [ 21 , 81 ]. Other sources of heterogeneity between studies may include whether the case sampling frame included only samples from culture positive LD patients. Similarly, the impact of type of sample, prospective vs. retrospective patients and sample libraries or serum panels for test performance was investigated wherever possible in the analysis. The control group samples in the captured studies ranged from groups of healthy individuals from endemic and non-endemic areas to controls with diseases known to cross-react with LD diagnostic assays. Despite this, most studies reported a consistently high specificity for LD regardless of the composition of the control group and where there were differences (Tables 2 – 4 ), these were not statistically significant in most cases.
There was a wide range of assays identified in this systematic review including those assays that employed whole-cell sonicates mainly from B . burgdorferi B31 or other North American isolates to recombinant proteins targeting antigens that are highly expressed in vivo e.g. VlsE. Some of the captured research indicates that the VlsE targets improve test performance [ 45 , 82 ]. Similarly the C6 peptide which is derived from the VlsE lipoprotein has shown equivalent or better sensitivity compared to the WCS ELISAs in this systematic review, improved specificity for patients with often cross-reactive diseases and may also be used to identify some species of Borrelia acquired in Europe [ 47 , 73 , 74 , 82 – 84 ]. Subjectivity and inconsistency of the criteria used to evaluate western blot results has been noted as a source of confusion for patients and physicians in the interpretation of diagnostic results [ 85 , 86 ]. In studies where the CDC western blot interpretation was paired with different criteria, some showed gains in sensitivity with alternate criteria, but this was usually accompanied by a reduced specificity below an acceptable level [ 46 ].
Direct detection of B . burgdorferi from LD patient samples continues to be a challenge. B . burgdorferi requires culture in a complex medium for 8 to 12 weeks before the culture is considered negative, which makes this approach unsuitable in a clinical setting. Recent studies have attempted to improve the utility of culture by changing the protocol, for example, use of a 60 ml of BSK in a closed tube, incubated at 32–33°C for 8–12 weeks [ 58 , 59 ]. Another study used 15 ml and 2 ml starter cultures, then at day six seeded a long term culture in a caliper jar with 15 ml of fresh BSK for up to 16 weeks at 34°C [ 57 ]. Variations that had positive effects on culture growth included adding serum, a reducing agent and rifampicin [ 57 – 59 ]. The use of PCR to confirm bacterial isolation improves the sensitivity compared to visual confirmation by staining with acridine-orange and using dark-field microscopy or fluorescent microscopy [ 69 ]. The specimen, stage of LD and the laboratory technician’s experience has an effect on the likelihood of obtaining a successful B . burgdorferi culture. In early LD a biopsy sample from an EM lesion taken within the first week of symptoms has the highest sensitivity, whereas early disseminated infections have a higher sensitivity if isolation is attempted on large volume plasma samples [ 59 , 69 ].
Bacterial isolation has had limited success with late manifestations of LD and with cerebrospinal fluid and synovial fluid samples [ 87 , 88 ]. Research continues to focus on improving the sensitivity and speed of culture. Recent papers claiming major breakthroughs for B . burgdorferi isolation have failed validation [ 57 ] or could not be replicated [ 65 ] by others [ 64 , 67 ]. PCR for detection of B . burgdorferi DNA in LD patient samples is affected by many of the same limitations as culture with the exception that results may be obtained faster and PCR may be more sensitive in samples with a low concentration of B . burgdorferi . The variability of methodologies, gene targets and primers from study to study continue to impact the interpretation of the PCR results [ 59 , 61 , 62 ]. Overall, the sensitivities of PCR studies conducted in North America were lower than those that employed a two-tiered serology diagnostic protocol [ 59 , 61 , 62 ]. Due to the above limitations, bacterial isolation and PCR are not routinely used as diagnostic tools in clinical practise, although bacterial isolation is considered the gold standard to confirm diagnosis.
From the peer-reviewed literature we identified validation data from only a small proportion of licensed assays and for a number of “in house” tests which are used by several laboratories across North America. The performance of “in house” tests cannot be validated or critiqued as the composition of the test is not always publically available or evaluated in the peer-reviewed literature, thus comparing their performance to licensed tests is less informative. In studies looking at the variable performance of diagnostic testing schemes across laboratories it has been demonstrated that deviations from recommended diagnostic schemes often lead to a decrease in specificity and discordant results with approved testing schemes [ 89 ]. Thus, the performance of these “in house” assays and some of the older commercial assays have not been evaluated against well characterised panels of serum from patients with the full spectrum of LD clinical symptoms, with appropriate numbers of healthy controls and patients with look-alike diseases [ 32 ].
Future work on diagnostic tests for LD includes continued improvement in the sensitivity of all tests, particularly for early LD samples and the ability to distinguish between active infection and previous infections. On-going work into new immunoassay techniques and combinations of antigen targets that may help inform disease stage will hopefully improve LD diagnostics in the future [ 60 , 79 , 90 ]. Development of point-of care tests that do not require highly specialized technical skills and subjective interpretation of the results would help address some of the criticisms of immunoblot techniques. This systematic review summarizes research in North America on the accuracy of diagnostic tests for LD conducted since 1995. The performance of the commercially available Immunetics® C6 B . burgdorferi ELISA™ shows the most promise as a possible standalone test or as part of a two-tiered test protocol; however it did not overcome the low sensitivity of LD diagnostic tests in patients with early LD. Addressing this shortcoming is a significant challenge to improving LD diagnostics.
Supporting Information
Data availability.
All relevant data are within the paper and its Supporting Information files and S3 contains the datasets for each table.
Funding Statement
The authors received no funding for this work.
- 1. Nelson CA, Saha S, Kugeler KJ, Delorey MJ, Shankar MB, Hinckley AF, et al. Incidence of Clinician-Diagnosed Lyme Disease, United States, 2005–2010. Emerg Infect Dis. 2015;21: 1625–1631. 10.3201/eid2109.150417 [ DOI ] [ PMC free article ] [ PubMed ] [ Google Scholar ]
- 2. Ogden NH, Lindsay LR, Morshed M, Sockett PN, Artsob H. The emergence of Lyme disease in Canada. CMAJ. 2009;180: 1221–1224. 10.1503/cmaj.080148 [ DOI ] [ PMC free article ] [ PubMed ] [ Google Scholar ]
- 3. Steere AC, Malawista SE, Snydman DR, Shope RE, Andiman WA, Ross MR, et al. Lyme arthritis: an epidemic of oligoarticular arthritis in children and adults in three connecticut communities. Arthritis Rheum. 1977;20: 7–17. [ DOI ] [ PubMed ] [ Google Scholar ]
- 4. Scrimenti RJ. Erythema chronicum migrans. Arch Dermatol. 1970;102: 104–105. [ PubMed ] [ Google Scholar ]
- 5. Onyett H, Canadian Paediatric Society, Infectious Diseases and Immunization Committee. Lyme disease in Canada: Focus on children. Paediatr Child Health. 2014;19: 379–388. [ PMC free article ] [ PubMed ] [ Google Scholar ]
- 6. Stanek G, Wormser GP, Gray J, Strle F. Lyme borreliosis. Lancet. 2012;379: 461–473. 10.1016/S0140-6736(11)60103-7 [ DOI ] [ PubMed ] [ Google Scholar ]
- 7. Centers for Disease Control and Prevention (CDC). Signs and Symptoms of Untreated Lyme Disease. 2015. Available: http://www.cdc.gov/lyme/signs_symptoms/
- 8. Pritt BS, Mead PS, Johnson DK, Neitzel DF, Respicio-Kingry LB, Davis JP, et al. Identification of a novel pathogenic Borrelia species causing Lyme borreliosis with unusually high spirochaetaemia: a descriptive study. Lancet Infect Dis. 2016. [ DOI ] [ PMC free article ] [ PubMed ] [ Google Scholar ]
- 9. Nelder MP, Russell C, Lindsay LR, Dhar B, Patel SN, Johnson S, et al. Population-based passive tick surveillance and detection of expanding foci of blacklegged ticks Ixodes scapularis and the Lyme disease agent Borrelia burgdorferi in Ontario, Canada. PLoS One. 2014;9: e105358 10.1371/journal.pone.0105358 [ DOI ] [ PMC free article ] [ PubMed ] [ Google Scholar ]
- 10. Stone BL, Russart NM, Gaultney RA, Floden AM, Vaughan JA, Brissette CA. The Western progression of lyme disease: infectious and Nonclonal Borrelia burgdorferi Sensu Lato populations in Grand Forks County, North Dakota. Appl Environ Microbiol. 2015;81: 48–58. 10.1128/AEM.02422-14 [ DOI ] [ PMC free article ] [ PubMed ] [ Google Scholar ]
- 11. Stanek G, Fingerle V, Hunfeld KP, Jaulhac B, Kaiser R, Krause A, et al. Lyme borreliosis: clinical case definitions for diagnosis and management in Europe. Clin Microbiol Infect. 2011;17: 69–79. 10.1111/j.1469-0691.2010.03175.x [ DOI ] [ PubMed ] [ Google Scholar ]
- 12. Eisen RJ, Piesman J, Zielinski-Gutierrez E, Eisen L. What do we need to know about disease ecology to prevent Lyme disease in the northeastern United States? J Med Entomol. 2012;49: 11–22. [ DOI ] [ PubMed ] [ Google Scholar ]
- 13. Hamer SA, Tsao JI, Walker ED, Hickling GJ. Invasion of the lyme disease vector Ixodes scapularis: implications for Borrelia burgdorferi endemicity. Ecohealth. 2010;7: 47–63. 10.1007/s10393-010-0287-0 [ DOI ] [ PubMed ] [ Google Scholar ]
- 14. Koffi JK, Leighton PA, Pelcat Y, Trudel L, Lindsay LR, Milord F, et al. Passive surveillance for I. scapularis ticks: enhanced analysis for early detection of emerging Lyme disease risk. J Med Entomol. 2012;49: 400–409. [ DOI ] [ PubMed ] [ Google Scholar ]
- 15. Brownstein JS, Holford TR, Fish D. Effect of Climate Change on Lyme Disease Risk in North America. Ecohealth. 2005;2: 38–46. 10.1007/s10393-004-0139-x [ DOI ] [ PMC free article ] [ PubMed ] [ Google Scholar ]
- 16. Ogden NH, Maarouf A, Barker IK, Bigras-Poulin M, Lindsay LR, Morshed MG, et al. Climate change and the potential for range expansion of the Lyme disease vector Ixodes scapularis in Canada. Int J Parasitol. 2006;36: 63–70. 10.1016/j.ijpara.2005.08.016 [ DOI ] [ PubMed ] [ Google Scholar ]
- 17. Lindsay LR, Bernat K, Dibernardo A. Laboratory diagnostics for Lyme disease. CCDR. 2014;40. [ DOI ] [ PMC free article ] [ PubMed ] [ Google Scholar ]
- 18. Nelson C, Hojvat S, Johnson B, Petersen J, Schriefer M, Beard CB, et al. Concerns regarding a new culture method for Borrelia burgdorferi not approved for the diagnosis of Lyme disease. MMWR Morb Mortal Wkly Rep. 2014;63: 333 [ PMC free article ] [ PubMed ] [ Google Scholar ]
- 19. Health Canada Medical Devices Branch. MDALL—Your reference tool for licensed medical devices in Canada. 2015. Available: http://webprod5.hc-sc.gc.ca/mdll-limh/index-eng.jsp
- 20. US Food and Drug Administration. Devices@FDA. 2015. Available: http://www.accessdata.fda.gov/scripts/cdrh/devicesatfda/index.cfm
- 21. Centers for Disease Control and Prevention (CDC). Lyme Disease- Two-step Laboratory Testing Process. 2015. Available: http://www.cdc.gov/lyme/diagnosistesting/labtest/twostep/index.html
- 22. Marques AR. Laboratory diagnosis of Lyme disease: advances and challenges. Infect Dis Clin North Am. 2015;29: 295–307. 10.1016/j.idc.2015.02.005 [ DOI ] [ PMC free article ] [ PubMed ] [ Google Scholar ]
- 23. Centers for Disease Control and Prevention (CDC). Recommendations for test performance and interpretation from the Second National Conference on Serologic Diagnosis of Lyme Disease. MMWR Morb Mortal Wkly Rep. 1995;44: 590–591. [ PubMed ] [ Google Scholar ]
- 24. Dumler JS. Molecular diagnosis of Lyme disease: review and meta-analysis. Mol Diagn. 2001;6: 1–11. 10.1054/modi.2001.21898 [ DOI ] [ PubMed ] [ Google Scholar ]
- 25. Leeflang MM, Ang CW, Berkhout J, Bijlmer HA, Van Bortel W, Brandenburg AH, et al. The diagnostic accuracy of serological tests for Lyme borreliosis in Europe: a systematic review and meta-analysis. BMC Infect Dis. 2016;16: 140-016-1468-4. [ DOI ] [ PMC free article ] [ PubMed ] [ Google Scholar ]
- 26. Greig J, Young I, Harding S, Mascarenhas M, Waddell LA, Michel P. A Scoping Review of Research on Lyme Disease Published up to September 2013. Public Health Agency of Canada. Available upon request.
- 27. Whiting PF, Rutjes AW, Westwood ME, Mallett S, QUADAS-2 Steering Group. A systematic review classifies sources of bias and variation in diagnostic test accuracy studies. J Clin Epidemiol. 2013;66: 1093–1104. 10.1016/j.jclinepi.2013.05.014 [ DOI ] [ PubMed ] [ Google Scholar ]
- 28. Whiting PF, Rutjes AW, Westwood ME, Mallett S, Deeks JJ, Reitsma JB, et al. QUADAS-2: a revised tool for the quality assessment of diagnostic accuracy studies. Ann Intern Med. 2011;155: 529–536. 10.7326/0003-4819-155-8-201110180-00009 [ DOI ] [ PubMed ] [ Google Scholar ]
- 29. Whiting P, Harbord R, de Salis I, Egger M, Sterne J. Evidence-based diagnosis. J Health Serv Res Policy. 2008;13 Suppl 3: 57–63. [ DOI ] [ PubMed ] [ Google Scholar ]
- 30. Viswanathan M, Ansari MT, Berkman ND, Chang S, Hartling L, McPheeters M, et al. Assessing the Risk of Bias of Individual Studies in Systematic Reviews of Health Care Interventions. In: Anonymous Methods Guide for Effectiveness and Comparative Effectiveness Reviews. Rockville (MD); 2008. [ PubMed ]
- 31. Berkman ND, Lohr KN, Ansari MT, Balk EM, Kane R, McDonagh M, et al. Grading the strength of a body of evidence when assessing health care interventions: an EPC update. J Clin Epidemiol. 2015;68: 1312–1324. 10.1016/j.jclinepi.2014.11.023 [ DOI ] [ PubMed ] [ Google Scholar ]
- 32. Molins CR, Sexton C, Young JW, Ashton LV, Pappert R, Beard CB, et al. Collection and characterization of samples for establishment of a serum repository for lyme disease diagnostic test development and evaluation. J Clin Microbiol. 2014;52: 3755–3762. 10.1128/JCM.01409-14 [ DOI ] [ PMC free article ] [ PubMed ] [ Google Scholar ]
- 33. Yu Z, Carter JM, Sigal LH, Stein S. Multi-well ELISA based on independent peptide antigens for antibody capture. Application to Lyme disease serodiagnosis. J Immunol Methods. 1996;198: 25–33. [ DOI ] [ PubMed ] [ Google Scholar ]
- 34. Liang FT, Steere AC, Marques AR, Johnson BJ, Miller JN, Philipp MT. Sensitive and specific serodiagnosis of Lyme disease by enzyme-linked immunosorbent assay with a peptide based on an immunodominant conserved region of Borrelia burgdorferi vlsE. J Clin Microbiol. 1999;37: 3990–3996. [ DOI ] [ PMC free article ] [ PubMed ] [ Google Scholar ]
- 35. Mogilyansky E, Loa CC, Adelson ME, Mordechai E, Tilton RC. Comparison of Western immunoblotting and the C6 Lyme antibody test for laboratory detection of Lyme disease. Clin Diagn Lab Immunol. 2004;11: 924–929. 10.1128/CDLI.11.5.924-929.2004 [ DOI ] [ PMC free article ] [ PubMed ] [ Google Scholar ]
- 36. Vyas J. Lyme disease. 2014. Available: https://www.nlm.nih.gov/medlineplus/ency/article/001319.htm
- 37. Centers for Disease Control and Prevention (CDC). Post-Treatment Lyme Disease Syndrome. 2015. Available: http://www.cdc.gov/lyme/postlds/
- 38. Feder HM Jr, Johnson BJ, O'Connell S, Shapiro ED, Steere AC, Wormser GP, et al. A critical appraisal of "chronic Lyme disease". N Engl J Med. 2007;357: 1422–1430. 10.1056/NEJMra072023 [ DOI ] [ PubMed ] [ Google Scholar ]
- 39. Harbord RM, Whiting PF. metandi: Meta-analysis of diagnostic accuracy using hierarchical logistic regression. SJ. 2009;9: 211–229. [ Google Scholar ]
- 40. Rutter CM, Gatsonis CA. A hierarchical regression approach to meta-analysis of diagnostic test accuracy evaluations. Stat Med. 2001;20: 2865–2884. [ DOI ] [ PubMed ] [ Google Scholar ]
- 41. Reitsma JB, Glas AS, Rutjes AW, Scholten RJ, Bossuyt PM, Zwinderman AH. Bivariate analysis of sensitivity and specificity produces informative summary measures in diagnostic reviews. J Clin Epidemiol. 2005;58: 982–990. 10.1016/j.jclinepi.2005.02.022 [ DOI ] [ PubMed ] [ Google Scholar ]
- 42. Takwoingi Y, Guo B, Riley RD, Deeks JJ. Performance of methods for meta-analysis of diagnostic test accuracy with few studies or sparse data. Stat Methods Med Res. 2015. [ DOI ] [ PMC free article ] [ PubMed ] [ Google Scholar ]
- 43. Porwancher RB, Hagerty CG, Fan J, Landsberg L, Johnson BJ, Kopnitsky M, et al. Multiplex immunoassay for Lyme disease using VlsE1-IgG and pepC10-IgM antibodies: improving test performance through bioinformatics. Clin Vaccine Immunol. 2011;18: 851–859. 10.1128/CVI.00409-10 [ DOI ] [ PMC free article ] [ PubMed ] [ Google Scholar ]
- 44. Magnarelli LA, Fikrig E, Padula SJ, Anderson JF, Flavell RA. Use of recombinant antigens of Borrelia burgdorferi in serologic tests for diagnosis of lyme borreliosis. J Clin Microbiol. 1996;34: 237–240. [ DOI ] [ PMC free article ] [ PubMed ] [ Google Scholar ]
- 45. Branda JA, Aguero-Rosenfeld ME, Ferraro MJ, Johnson BJ, Wormser GP, Steere AC. 2-tiered antibody testing for early and late Lyme disease using only an immunoglobulin G blot with the addition of a VlsE band as the second-tier test. Clin Infect Dis. 2010;50: 20–26. 10.1086/648674 [ DOI ] [ PubMed ] [ Google Scholar ]
- 46. Tilton RC, Sand MN, Manak M. The western immunoblot for Lyme disease: determination of sensitivity, specificity, and interpretive criteria with use of commercially available performance panels. Clin Infect Dis. 1997;25 Suppl 1: S31–4. [ DOI ] [ PubMed ] [ Google Scholar ]
- 47. Wormser GP, Schriefer M, Aguero-Rosenfeld ME, Levin A, Steere AC, Nadelman RB, et al. Single-tier testing with the C6 peptide ELISA kit compared with two-tier testing for Lyme disease. Diagn Microbiol Infect Dis. 2013;75: 9–15. 10.1016/j.diagmicrobio.2012.09.003 [ DOI ] [ PMC free article ] [ PubMed ] [ Google Scholar ]
- 48. Zwinderman AH, Bossuyt PM. We should not pool diagnostic likelihood ratios in systematic reviews. Stat Med. 2008;27: 687–697. 10.1002/sim.2992 [ DOI ] [ PubMed ] [ Google Scholar ]
- 49. Qiu B, Brunner M, Zhang G, Sigal L, Stein S. Selection of continuous epitope sequences and their incorporation into poly(ethylene glycol)-peptide conjugates for use in serodiagnostic immunoassays: application to Lyme disease. Biopolymers. 2000;55: 319–333. [ DOI ] [ PubMed ] [ Google Scholar ]
- 50. Pavia CS, Wormser GP, Bittker S, Cooper D. An indirect hemagglutination antibody test to detect antibodies to Borrelia burgdorferi in patients with Lyme disease. J Microbiol Methods. 2000;40: 163–173. [ DOI ] [ PubMed ] [ Google Scholar ]
- 51. Gomes-Solecki MJ, Wormser GP, Schriefer M, Neuman G, Hannafey L, Glass JD, et al. Recombinant assay for serodiagnosis of Lyme disease regardless of OspA vaccination status. J Clin Microbiol. 2002;40: 193–197. 10.1128/JCM.40.1.193-197.2002 [ DOI ] [ PMC free article ] [ PubMed ] [ Google Scholar ]
- 52. Fawcett PT, Rose CD, Gibney KM, Doughty RA. Comparison of immunodot and western blot assays for diagnosing Lyme borreliosis. Clin Diagn Lab Immunol. 1998;5: 503–506. [ DOI ] [ PMC free article ] [ PubMed ] [ Google Scholar ]
- 53. Ledue TB, Collins MF, Young J, Schriefer ME. Evaluation of the recombinant VlsE-based liaison chemiluminescence immunoassay for detection of Borrelia burgdorferi and diagnosis of Lyme disease. Clin Vaccine Immunol. 2008;15: 1796–1804. 10.1128/CVI.00195-08 [ DOI ] [ PMC free article ] [ PubMed ] [ Google Scholar ]
- 54. Jobe DA, Lovrich SD, Asp KE, Mathiason MA, Albrecht SE, Schell RF, et al. Significantly improved accuracy of diagnosis of early Lyme disease by peptide enzyme-linked immunosorbent assay based on the borreliacidal antibody epitope of Borrelia burgdorferi OspC. Clin Vaccine Immunol. 2008;15: 981–985. 10.1128/CVI.00079-08 [ DOI ] [ PMC free article ] [ PubMed ] [ Google Scholar ]
- 55. Trevejo RT, Krause PJ, Sikand VK, Schriefer ME, Ryan R, Lepore T, et al. Evaluation of two-test serodiagnostic method for early Lyme disease in clinical practice. J Infect Dis. 1999;179: 931–938. 10.1086/314663 [ DOI ] [ PubMed ] [ Google Scholar ]
- 56. Brissette CA, Rossmann E, Bowman A, Cooley AE, Riley SP, Hunfeld KP, et al. The borrelial fibronectin-binding protein RevA is an early antigen of human Lyme disease. Clin Vaccine Immunol. 2010;17: 274–280. 10.1128/CVI.00437-09 [ DOI ] [ PMC free article ] [ PubMed ] [ Google Scholar ]
- 57. Sapi E, Pabbati N, Datar A, Davies EM, Rattelle A, Kuo BA. Improved culture conditions for the growth and detection of Borrelia from human serum. Int J Med Sci. 2013;10: 362–376. 10.7150/ijms.5698 [ DOI ] [ PMC free article ] [ PubMed ] [ Google Scholar ]
- 58. Wormser GP, Nowakowski J, Nadelman RB, Bittker S, Cooper D, Pavia C. Improving the yield of blood cultures for patients with early Lyme disease. J Clin Microbiol. 1998;36: 296–298. [ DOI ] [ PMC free article ] [ PubMed ] [ Google Scholar ]
- 59. Liveris D, Schwartz I, McKenna D, Nowakowski J, Nadelman R, Demarco J, et al. Comparison of five diagnostic modalities for direct detection of Borrelia burgdorferi in patients with early Lyme disease. Diagn Microbiol Infect Dis. 2012;73: 243–245. 10.1016/j.diagmicrobio.2012.03.026 [ DOI ] [ PMC free article ] [ PubMed ] [ Google Scholar ]
- 60. Gomes-Solecki MJ, Wormser GP, Persing DH, Berger BW, Glass JD, Yang X, et al. A first-tier rapid assay for the serodiagnosis of Borrelia burgdorferi infection. Arch Intern Med. 2001;161: 2015–2020. [ DOI ] [ PubMed ] [ Google Scholar ]
- 61. Eshoo MW, Crowder CC, Rebman AW, Rounds MA, Matthews HE, Picuri JM, et al. Direct molecular detection and genotyping of Borrelia burgdorferi from whole blood of patients with early Lyme disease. PLoS One. 2012;7: e36825 10.1371/journal.pone.0036825 [ DOI ] [ PMC free article ] [ PubMed ] [ Google Scholar ]
- 62. Nocton JJ, Bloom BJ, Rutledge BJ, Persing DH, Logigian EL, Schmid CH, et al. Detection of Borrelia burgdorferi DNA by polymerase chain reaction in cerebrospinal fluid in Lyme neuroborreliosis. J Infect Dis. 1996;174: 623–627. [ DOI ] [ PubMed ] [ Google Scholar ]
- 63. Wang G, Iyer R, Bittker S, Cooper D, Small J, Wormser GP, et al. Variations in Barbour-Stoenner-Kelly culture medium modulate infectivity and pathogenicity of Borrelia burgdorferi clinical isolates. Infect Immun. 2004;72: 6702–6706. 10.1128/IAI.72.11.6702-6706.2004 [ DOI ] [ PMC free article ] [ PubMed ] [ Google Scholar ]
- 64. Johnson BJ, Pilgard MA, Russell TM. Assessment of new culture method for detection of Borrelia species from serum of lyme disease patients. J Clin Microbiol. 2014;52: 721–724. 10.1128/JCM.01674-13 [ DOI ] [ PMC free article ] [ PubMed ] [ Google Scholar ]
- 65. Phillips SE, Mattman LH, Hulinska D, Moayad H. A proposal for the reliable culture of Borrelia burgdorferi from patients with chronic Lyme disease, even from those previously aggressively treated. Infection. 1998;26: 364–367. [ DOI ] [ PubMed ] [ Google Scholar ]
- 66. Tilton RC, Barden D, Sand M. Culture Borrelia burgdorferi. J Clin Microbiol. 2001;39: 2747 10.1128/JCM.39.7.2747.2001 [ DOI ] [ PMC free article ] [ PubMed ] [ Google Scholar ]
- 67. Marques AR, Stock F, Gill V. Evaluation of a new culture medium for Borrelia burgdorferi. J Clin Microbiol. 2000;38: 4239–4241. [ DOI ] [ PMC free article ] [ PubMed ] [ Google Scholar ]
- 68. Wormser GP, Bittker S, Cooper D, Nowakowski J, Nadelman RB, Pavia C. Comparison of the yields of blood cultures using serum or plasma from patients with early Lyme disease. J Clin Microbiol. 2000;38: 1648–1650. [ DOI ] [ PMC free article ] [ PubMed ] [ Google Scholar ]
- 69. Liveris D, Schwartz I, Bittker S, Cooper D, Iyer R, Cox ME, et al. Improving the yield of blood cultures from patients with early Lyme disease. J Clin Microbiol. 2011;49: 2166–2168. 10.1128/JCM.00350-11 [ DOI ] [ PMC free article ] [ PubMed ] [ Google Scholar ]
- 70. Liveris D, Wang G, Girao G, Byrne DW, Nowakowski J, McKenna D, et al. Quantitative detection of Borrelia burgdorferi in 2-millimeter skin samples of erythema migrans lesions: correlation of results with clinical and laboratory findings. J Clin Microbiol. 2002;40: 1249–1253. 10.1128/JCM.40.4.1249-1253.2002 [ DOI ] [ PMC free article ] [ PubMed ] [ Google Scholar ]
- 71. Gomes-Solecki MJ, Meirelles L, Glass J, Dattwyler RJ. Epitope length, genospecies dependency, and serum panel effect in the IR6 enzyme-linked immunosorbent assay for detection of antibodies to Borrelia burgdorferi. Clin Vaccine Immunol. 2007;14: 875–879. 10.1128/CVI.00122-07 [ DOI ] [ PMC free article ] [ PubMed ] [ Google Scholar ]
- 72. Burbelo PD, Issa AT, Ching KH, Cohen JI, Iadarola MJ, Marques A. Rapid, simple, quantitative, and highly sensitive antibody detection for lyme disease. Clin Vaccine Immunol. 2010;17: 904–909. 10.1128/CVI.00476-09 [ DOI ] [ PMC free article ] [ PubMed ] [ Google Scholar ]
- 73. Branda JA, Strle F, Strle K, Sikand N, Ferraro MJ, Steere AC. Performance of United States serologic assays in the diagnosis of Lyme borreliosis acquired in Europe. Clin Infect Dis. 2013;57: 333–340. 10.1093/cid/cit235 [ DOI ] [ PMC free article ] [ PubMed ] [ Google Scholar ]
- 74. Sillanpaa H, Lahdenne P, Sarvas H, Arnez M, Steere A, Peltomaa M, et al. Immune responses to borrelial VlsE IR6 peptide variants. Int J Med Microbiol. 2007;297: 45–52. 10.1016/j.ijmm.2006.09.001 [ DOI ] [ PubMed ] [ Google Scholar ]
- 75. Hinckley AF, Connally NP, Meek JI, Johnson BJ, Kemperman MM, Feldman KA, et al. Lyme disease testing by large commercial laboratories in the United States. Clin Infect Dis. 2014;59: 676–681. 10.1093/cid/ciu397 [ DOI ] [ PMC free article ] [ PubMed ] [ Google Scholar ]
- 76. Maraspin V, Ogrinc K, Ruzic-Sabljic E, Lotric-Furlan S, Strle F. Isolation of Borrelia burgdorferi sensu lato from blood of adult patients with borrelial lymphocytoma, Lyme neuroborreliosis, Lyme arthritis and acrodermatitis chronica atrophicans. Infection. 2011;39: 35–40. 10.1007/s15010-010-0062-8 [ DOI ] [ PubMed ] [ Google Scholar ]
- 77. Fallon BA, Pavlicova M, Coffino SW, Brenner C. A comparison of lyme disease serologic test results from 4 laboratories in patients with persistent symptoms after antibiotic treatment. Clin Infect Dis. 2014;59: 1705–1710. 10.1093/cid/ciu703 [ DOI ] [ PubMed ] [ Google Scholar ]
- 78. Stanek G, Lusa L, Ogrinc K, Markowicz M, Strle F. Intrathecally produced IgG and IgM antibodies to recombinant VlsE, VlsE peptide, recombinant OspC and whole cell extracts in the diagnosis of Lyme neuroborreliosis. Med Microbiol Immunol. 2014;203: 125–132. 10.1007/s00430-013-0322-1 [ DOI ] [ PubMed ] [ Google Scholar ]
- 79. Dessau RB, Moller JK, Kolmos B, Henningsson AJ. Multiplex assay (Mikrogen recomBead) for detection of serum IgG and IgM antibodies to 13 recombinant antigens of Borrelia burgdorferi sensu lato in patients with neuroborreliosis: the more the better? J Med Microbiol. 2015;64: 224–231. 10.1099/jmm.0.000009 [ DOI ] [ PubMed ] [ Google Scholar ]
- 80. Henningsson AJ, Christiansson M, Tjernberg I, Lofgren S, Matussek A. Laboratory diagnosis of Lyme neuroborreliosis: a comparison of three CSF anti-Borrelia antibody assays. Eur J Clin Microbiol Infect Dis. 2014;33: 797–803. 10.1007/s10096-013-2014-6 [ DOI ] [ PMC free article ] [ PubMed ] [ Google Scholar ]
- 81. Canadian Public Health Laboratory Network. The laboratory diagnosis of Lyme borreliosis: Guidelines from the Canadian Public Health Laboratory Network. Can J Infect Dis Med Microbiol. 2007;18: 145–148. [ DOI ] [ PMC free article ] [ PubMed ] [ Google Scholar ]
- 82. Branda JA, Linskey K, Kim YA, Steere AC, Ferraro MJ. Two-tiered antibody testing for Lyme disease with use of 2 enzyme immunoassays, a whole-cell sonicate enzyme immunoassay followed by a VlsE C6 peptide enzyme immunoassay. Clin Infect Dis. 2011;53: 541–547. 10.1093/cid/cir464 [ DOI ] [ PubMed ] [ Google Scholar ]
- 83. Wormser GP, Tang AT, Schimmoeller NR, Bittker S, Cooper D, Visintainer P, et al. Utility of serodiagnostics designed for use in the United States for detection of Lyme borreliosis acquired in Europe and vice versa. Med Microbiol Immunol. 2014;203: 65–71. 10.1007/s00430-013-0315-0 [ DOI ] [ PubMed ] [ Google Scholar ]
- 84. Bacon RM, Biggerstaff BJ, Schriefer ME, Gilmore RD Jr, Philipp MT, Steere AC, et al. Serodiagnosis of Lyme disease by kinetic enzyme-linked immunosorbent assay using recombinant VlsE1 or peptide antigens of Borrelia burgdorferi compared with 2-tiered testing using whole-cell lysates. J Infect Dis. 2003;187: 1187–1199. 10.1086/374395 [ DOI ] [ PMC free article ] [ PubMed ] [ Google Scholar ]
- 85. Binnicker MJ, Jespersen DJ, Harring JA, Rollins LO, Bryant SC, Beito EM. Evaluation of two commercial systems for automated processing, reading, and interpretation of Lyme borreliosis Western blots. J Clin Microbiol. 2008;46: 2216–2221. 10.1128/JCM.00200-08 [ DOI ] [ PMC free article ] [ PubMed ] [ Google Scholar ]
- 86. Schoen RT. Editorial commentary: better laboratory testing for Lyme disease: no more Western blot. Clin Infect Dis. 2013;57: 341–343. 10.1093/cid/cit238 [ DOI ] [ PubMed ] [ Google Scholar ]
- 87. Nowakowski J, McKenna D, Nadelman RB, Bittker S, Cooper D, Pavia C, et al. Blood cultures for patients with extracutaneous manifestations of Lyme disease in the United States. Clin Infect Dis. 2009;49: 1733–1735. 10.1086/648076 [ DOI ] [ PubMed ] [ Google Scholar ]
- 88. Cerar T, Ogrinc K, Cimperman J, Lotric-Furlan S, Strle F, Ruzic-Sabljic E. Validation of cultivation and PCR methods for diagnosis of Lyme neuroborreliosis. J Clin Microbiol. 2008;46: 3375–3379. 10.1128/JCM.00410-08 [ DOI ] [ PMC free article ] [ PubMed ] [ Google Scholar ]
- 89. Fallon BA, Pavlicova M, Coffino SW, Brenner C. A comparison of lyme disease serologic test results from 4 laboratories in patients with persistent symptoms after antibiotic treatment. Clin Infect Dis. 2014;59: 1705–1710. 10.1093/cid/ciu703 [ DOI ] [ PubMed ] [ Google Scholar ]
- 90. Lahey LJ, Panas MW, Mao R, Delanoy M, Flanagan JJ, Binder SR, et al. Development of a Multiantigen Panel for Improved Detection of Borrelia burgdorferi Infection in Early Lyme Disease. J Clin Microbiol. 2015;53: 3834–3841. 10.1128/JCM.02111-15 [ DOI ] [ PMC free article ] [ PubMed ] [ Google Scholar ]
Associated Data
This section collects any data citations, data availability statements, or supplementary materials included in this article.
Supplementary Materials
Data availability statement.
- View on publisher site
- PDF (2.6 MB)
- Collections
Similar articles
Cited by other articles, links to ncbi databases.
- Download .nbib .nbib
- Format: AMA APA MLA NLM
Add to Collections
Office of the Vice Provost for Research
Find Collaborators
Meaningful relationships are the foundation of successful, interdisciplinary research.
Faculty Profiles
Faculty Profiles is a search directory for Tufts faculty profiles which include bios, publications, research areas, and more.
Explore Tufts' scholarly activities using Dimensions, a research knowledge base.
Corporate Connections
Collaborations outside of academia are essential to Tufts success.
Tech Transfer and Industry Collaboration
Connect with Corporations or Foundations
Tufts Facilities & Technologies
Find research facilities and resources that are available at Tufts and connect with the experts who support them.
Search Core Facilities & Resources
Search Technologies for Licensing
Support Functions
Meet the teams that support research both within the OVPR and beyond.
Connect with Support Functions
Contact the OVPR
Tufts Centers & Institutes
Tufts University is home to more than 45 interdisciplinary centers and institutes with expertise on a broad range of topics.
New ‘Subway Map’ of Lyme Disease Pathways Identifies Potential New Treatment Targets
Thursday, october, 19th, 2023.
The model, created by Tufts researchers, showed that two existing drugs demonstrate potential for more selective therapeutic options
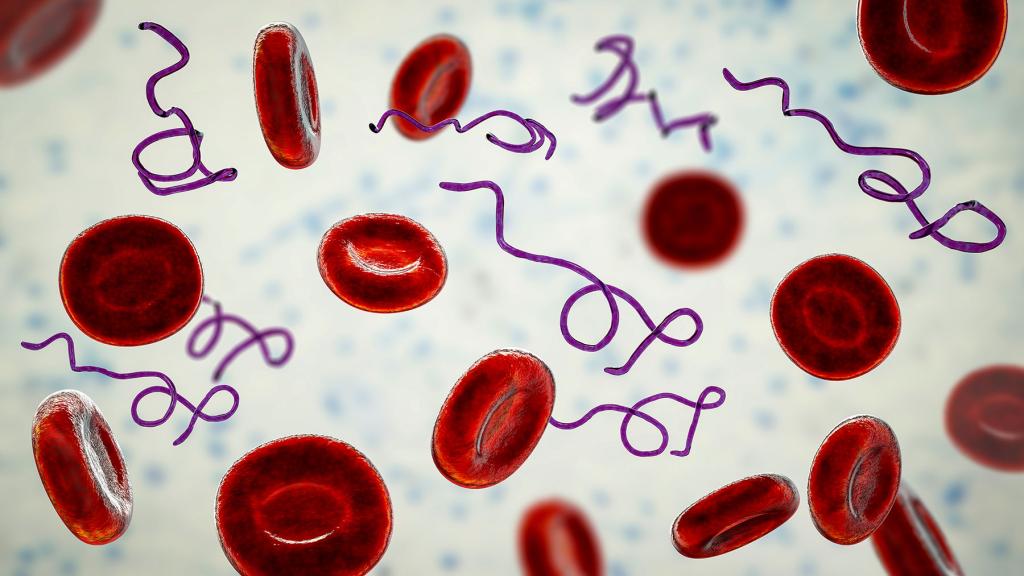
By Julie Rafferty
Scientists at Tufts University School of Medicine and the Graduate School of Biomedical Sciences (GSBS) have developed a genome-scale metabolic model or “subway map” of key metabolic activities of the bacterium that causes Lyme disease. Using this map, they have successfully identified two compounds that selectively target routes only used by Lyme disease to infect a host. Their research was published October 19 in the journal mSystems .
While neither medication is a viable treatment for Lyme because they have numerous side effects, the successful use of the computational “subway map” to predict drug targets and possible existing treatments demonstrates that it may be possible to develop micro-substances that only block Lyme disease while leaving other helpful bacteria untouched.
Genome-scale metabolic models (GEMs) collect all known metabolic information on a biological system, including the genes, enzymes, metabolites, and other information. These models use big data and machine learning to help scientists understand molecular mechanisms, make predictions, and identify new processes that might be previously unknown and even counter-intuitive to known biological processes.
Micro-Substances to Target Bacterium
Currently, Lyme disease is treated with broad-spectrum antibiotics that kill the Lyme bacterium Borrelia burgdorferi, but simultaneously also kill a wide range of the other bacteria that inhabit a host’s microbiome and perform many helpful functions. Some people with chronic Lyme symptoms or recurring Lyme disease take antibiotics for years, although it is against medical guidelines and there is no proof that it works.
“Most of the antibiotics we still use are based on discoveries that are decades old, and antibiotic resistance is an increasing problem across many bacterial diseases,” says Peter Gwynne , first author on the paper and research assistant professor of molecular biology and microbiology at Tufts University School of Medicine and the Tufts Lyme Disease Initiative . “There is a growing movement to find micro-substances that target a specific pathway in a single bacterium, rather than treating patients with broad spectrum antibiotics that wipe out the microbiome and cause antibiotic resistance.”
The two compounds identified using the “subway map” computational model are an anticancer drug with significant side effects that make it impractical to use in treating Lyme, and an asthma medication taken off the market because of its side effects. Both drugs identified by the model were tested in the lab and found to successfully kill Lyme bacteria—and only Lyme—in culture.
“The Lyme bacterium is a great test case for narrow spectrum drugs because it is so limited in what it can do and so highly dependent upon its environment. This leaves it vulnerable in ways other bacteria are not,” says Linden Hu , the Paul and Elaine Chervinsky Professor of Immunology, a professor of molecular biology and microbiology, and senior author on the study.
Speeding Treatment Discovery
Use of the computational model—which Gwynne and collaborators developed during COVID when they couldn’t work onsite in the lab—has the potential to enable scientists to skip some painstaking basic science steps and lead to swifter testing and development of more targeted treatments.
“We can now use this model to screen for similar compounds that don’t have the same toxicity of the anticancer and asthma medications but could potentially stop the same or another part of the Lyme disease process,” says Gwynne, a recent recipient of the Emerging Leader Award from the Bay Area Lyme Foundation .
Gwynne and Hu are conducting other research to determine whether people with chronic Lyme symptoms are still infected or are suffering from an immune malfunction that creates chronic symptoms . “I can imagine a day when people take a targeted Lyme treatment for two weeks rather than a broad-spectrum antibiotic, are tested and determined to be clear of the infection, and then take drugs to tame their immune response if chronic symptoms persist,” says Gwynne.
Gwynne says similar computational “subway maps” can be developed for other bacteria with relatively small genomes, such as those that cause the sexually transmitted diseases Syphilis and Chlamydia, and Rickettsia, which causes Rocky Mountain Spotted Fever. Gwynne’s team is looking at developing maps for some of these bacteria.
Citation: Research reported in this article was supported by the Bay Area Lyme Foundation and by the National Institute of Allergy and Infectious Diseases at the National Institutes of Health under award R01AI122286. Co-authors include Kee-Lee Stocks , a Ph.D. student at GSBS ; research assistant Aarya Pandit, E25, at GSBS; and former research assistant Elysse S. Karozichian, E23, at GSBS . Complete information on authors, funders, methodology, and conflicts of interest is available in the published paper.
Disclaimer: The content is solely the responsibility of the authors and does not necessarily represent the official views of the funders.
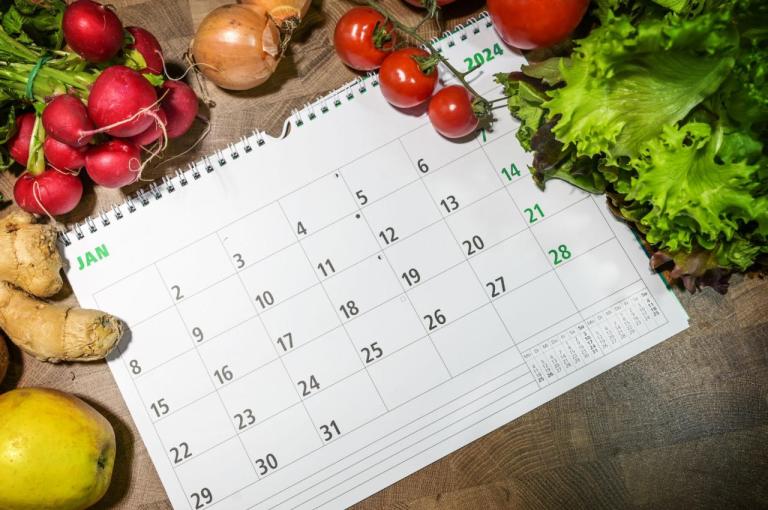
How to Keep Your Healthy New Year's Resolutions
- Download PDF
- Share X Facebook Email LinkedIn
- Permissions
As Lyme Disease Expands Its Reach, New Research Offers Hope
- Medical News & Perspectives Large Cohort Study Finds Possible Association Between Postural Orthostatic Tachycardia Syndrome and COVID-19 Vaccination but Far Stronger Link With SARS-CoV-2 Infection Rita Rubin, MA JAMA
- JAMA Patient Page What Is Alpha-Gal Syndrome? Fatema Mollah, MD; Mark A. Zacharek, MD; Mariel R. Benjamin, MD JAMA
Public health warnings about vector-borne diseases in the US are heating up. In a May editorial , scientists from the Centers for Disease Control and Prevention (CDC) made a plea for a vaccine against West Nile virus, which is spread by mosquitoes and has no treatment. By midsummer, the CDC reported that as many as 450 000 people in the US may have a meat allergy from the bite of the lone star tick, while other CDC scientists warned that mosquitoes are becoming resistant to the insecticides used in abatement efforts. Mosquitoes struck in August, when public health officials reported 11 cases of locally acquired dengue fever in Florida and Maryland’s first known case of locally acquired malaria in more than 40 years.
Read More About
Slomski A. As Lyme Disease Expands Its Reach, New Research Offers Hope. JAMA. 2023;330(12):1124–1126. doi:10.1001/jama.2023.15358
Manage citations:
© 2024
Cardiology in JAMA : Read the Latest
Browse and subscribe to JAMA Network podcasts!
Others Also Liked
Select your interests.
Customize your JAMA Network experience by selecting one or more topics from the list below.
- Academic Medicine
- Acid Base, Electrolytes, Fluids
- Allergy and Clinical Immunology
- American Indian or Alaska Natives
- Anesthesiology
- Anticoagulation
- Art and Images in Psychiatry
- Artificial Intelligence
- Assisted Reproduction
- Bleeding and Transfusion
- Caring for the Critically Ill Patient
- Challenges in Clinical Electrocardiography
- Climate and Health
- Climate Change
- Clinical Challenge
- Clinical Decision Support
- Clinical Implications of Basic Neuroscience
- Clinical Pharmacy and Pharmacology
- Complementary and Alternative Medicine
- Consensus Statements
- Coronavirus (COVID-19)
- Critical Care Medicine
- Cultural Competency
- Dental Medicine
- Dermatology
- Diabetes and Endocrinology
- Diagnostic Test Interpretation
- Digital Health
- Drug Development
- Electronic Health Records
- Emergency Medicine
- End of Life, Hospice, Palliative Care
- Environmental Health
- Equity, Diversity, and Inclusion
- Facial Plastic Surgery
- Gastroenterology and Hepatology
- Genetics and Genomics
- Genomics and Precision Health
- Global Health
- Guide to Statistics and Methods
- Hair Disorders
- Health Care Delivery Models
- Health Care Economics, Insurance, Payment
- Health Care Quality
- Health Care Reform
- Health Care Safety
- Health Care Workforce
- Health Disparities
- Health Inequities
- Health Policy
- Health Systems Science
- History of Medicine
- Hypertension
- Images in Neurology
- Implementation Science
- Infectious Diseases
- Innovations in Health Care Delivery
- JAMA Infographic
- Law and Medicine
- Leading Change
- Less is More
- LGBTQIA Medicine
- Lifestyle Behaviors
- Medical Coding
- Medical Devices and Equipment
- Medical Education
- Medical Education and Training
- Medical Journals and Publishing
- Mobile Health and Telemedicine
- Narrative Medicine
- Neuroscience and Psychiatry
- Notable Notes
- Nutrition, Obesity, Exercise
- Obstetrics and Gynecology
- Occupational Health
- Ophthalmology
- Orthopedics
- Otolaryngology
- Pain Medicine
- Palliative Care
- Pathology and Laboratory Medicine
- Patient Care
- Patient Information
- Performance Improvement
- Performance Measures
- Perioperative Care and Consultation
- Pharmacoeconomics
- Pharmacoepidemiology
- Pharmacogenetics
- Pharmacy and Clinical Pharmacology
- Physical Medicine and Rehabilitation
- Physical Therapy
- Physician Leadership
- Population Health
- Primary Care
- Professional Well-being
- Professionalism
- Psychiatry and Behavioral Health
- Public Health
- Pulmonary Medicine
- Regulatory Agencies
- Reproductive Health
- Research, Methods, Statistics
- Resuscitation
- Rheumatology
- Risk Management
- Scientific Discovery and the Future of Medicine
- Sexual Health
- Shared Decision Making and Communication
- Sleep Medicine
- Sports Medicine
- Stem Cell Transplantation
- Substance Use and Addiction Medicine
- Surgical Innovation
- Surgical Pearls
- Teachable Moment
- Technology and Finance
- The Art of JAMA
- The Arts and Medicine
- The Rational Clinical Examination
- Tobacco and e-Cigarettes
- Translational Medicine
- Trauma and Injury
- Treatment Adherence
- Ultrasonography
- Users' Guide to the Medical Literature
- Vaccination
- Venous Thromboembolism
- Veterans Health
- Women's Health
- Workflow and Process
- Wound Care, Infection, Healing
- Register for email alerts with links to free full-text articles
- Access PDFs of free articles
- Manage your interests
- Save searches and receive search alerts

A .gov website belongs to an official government organization in the United States.
A lock ( ) or https:// means you've safely connected to the .gov website. Share sensitive information only on official, secure websites.
- Signs and Symptoms
- How It Spreads
- Testing and Diagnosis
- Surveillance and Data
- Educational Materials
- Clinical Testing and Diagnosis
- Clinical Care and Treatment
- Continuing Education
- Clinical Resources
Related Topics:
- View All Home
- Vector-Borne Diseases
Clinical Care of Lyme Disease
- Early diagnosis and proper antibiotic treatment of Lyme disease is important and can help prevent more serious forms of the disease.
- The following treatment regimens reflect CDC’s interpretation of the most current data for four important manifestations of Lyme disease.
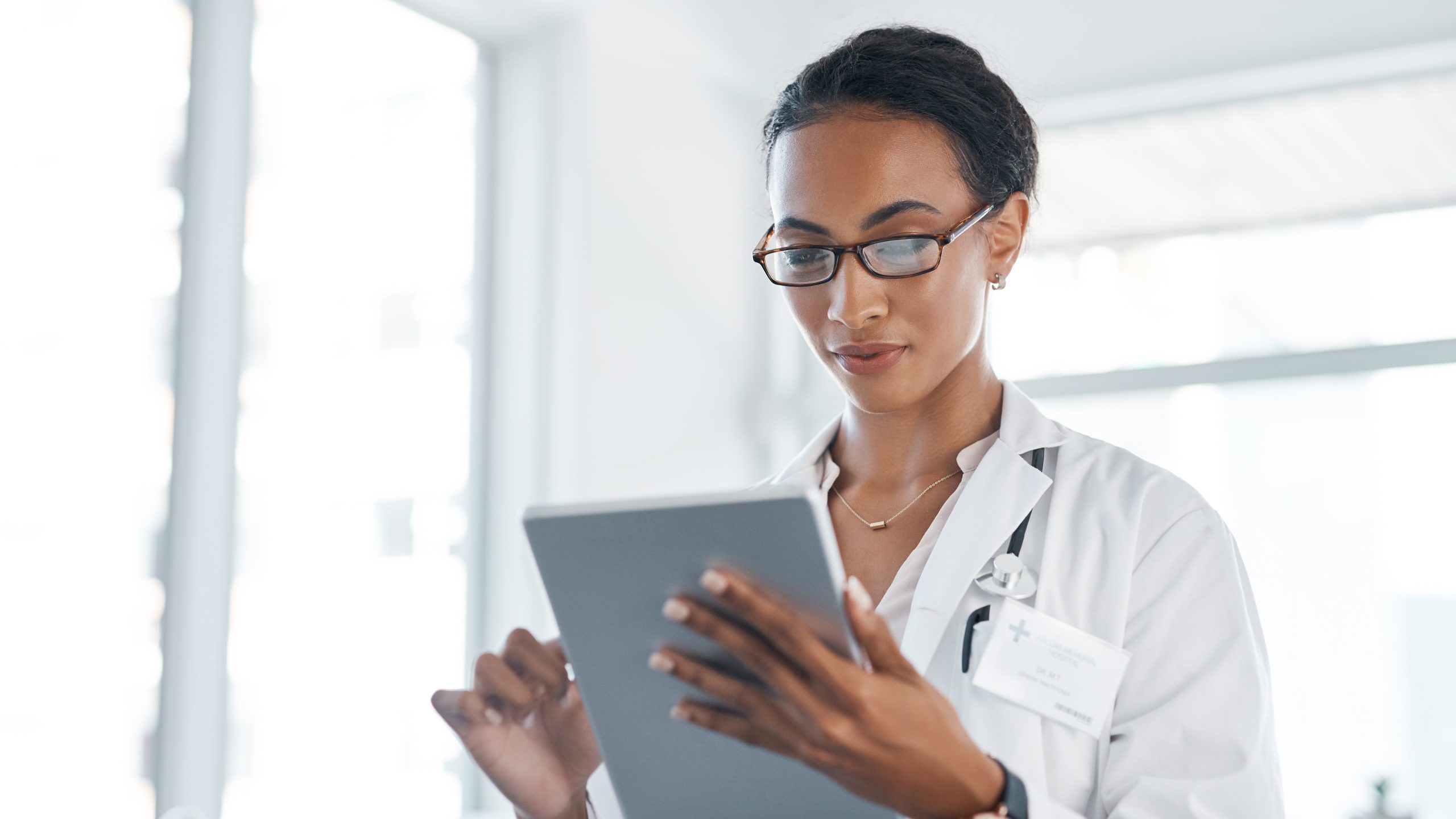
Treatment best practices
These regimens are consistent with guidance published by the by the Infectious Diseases Society of America, American Academy of Neurology, and American College of Rheumatology.
- Erythema migrans
- Neurologic Lyme disease
- Lyme carditis
- Lyme arthritis
Some patients report prolonged symptoms of pain, fatigue, or difficulty thinking even after treatment for Lyme disease. The state of the science relating to prolonged symptoms associated with Lyme disease is limited, emerging, and unsettled. Additional research is needed to better understand how to treat, manage, and support people with prolonged symptoms associated with Lyme disease.
Lyme Disease
Lyme disease is caused by Borrelia bacteria spread to people by the bite of an infected blacklegged tick.
For Everyone
Health care providers.
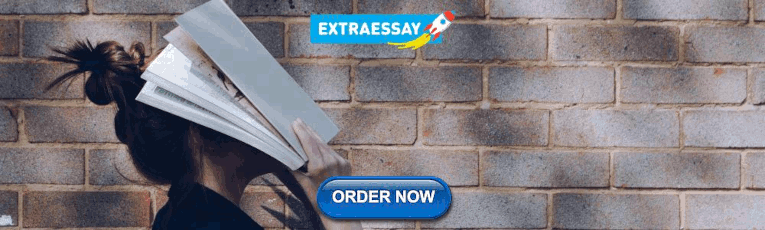
IMAGES
VIDEO
COMMENTS
Lyme Disease Research Center. The primary focus of the Johns Hopkins Lyme Disease Research Center (LDRC) is clinical translational research to advance the fundamental understanding of LD through the characterization of carefully constructed cohorts of LD patients and controls, as well as a clinical biorepository of blood and tissue biospecimens.
1 The findings of a systematic evidence map covering the whole range of research evidence on Lyme disease in humans (Stokes et al., 2017) were used to populate a number of focused, systematic evidence reviews, including this review on prevention methods. 2 CRD4201707151. 4 A list of papers excluded at full-text stage is available from the lead ...
This paper reviews the current literature on the diagnosis, etiology, risk factors, treatments and heterogeneity of patients with persistent symptoms of Lyme disease. The meaning and relevance of existing patient subgroups are discussed, as are future research priorities, including the need to develop illness biomarkers, elucidate the biologic ...
Lyme disease is caused by the spirochete, Borrelia burgdorferi sensu lato, a fastidious, microaerophilic bacterium that replicates slowly and requires special media to grow in the laboratory (Figure 1) [1]. The organism has been subclassified into several genomospecies, including B. burgdorferi sensu stricto, B. garinii, B. afzelii and others.
John Aucott, M.D., an infectious diseases specialist and the founding Director/physician of the Johns Hopkins Lyme Disease Research Center, is a world authority on this "post-treatment Lyme disease" (PTLD), a condition he helped define. Its symptoms are persistent, and can include fatigue, pain, weakness, brain fog, and sleep disorders.
Chronic Lyme disease is a broad, vaguely defined term that is used to describe patients with non-specific symptoms that are attributed to a presumed persistent Borrelia burgdorferi infection in ...
Furthermore, research into barriers of Lyme disease vaccine acceptability could be helpful in maximizing the potential for such a vaccine, if and when another comes to market. As new vaccine efficacy trials begin for Lyme disease, it is important to recognize factors that may lead to false conclusions of vaccine failure.
Time for a Different Approach to Lyme Disease and Long-Term Symptoms. Michael T. Melia, M.D., and Paul G. Auwaerter, M.D. The condition of most patients with Lyme dis-ease improves after initial antibiotic therapy; however, 10 to 20% of treated patients may have lingering symptoms of fatigue, musculoskeletal pains, disrupted sleep, and lack of ...
This study was funded by the Bay Area Lyme Foundation and supported with resources from the Tulane National Primate Research Center base grant of the National Institutes of Health, P51 OD011104 ...
In the "State of Lyme Disease Research," Center for Lyme Action makes 26 important recommendations. The paper is organized around the five research priorities from the 2019 NIH Strategic Plan for Tick-borne Disease Research, a comprehensive blueprint to guide a research program that can provide relief to patients across the United States ...
Current research efforts, partially supported by RFA-AI-19-037, include: Identifying B. burgdorferi antigens that could be used in next-generation Lyme disease vaccines. Studying the potential of a "chimerotope" vaccine, modeled after a recently approved canine vaccine for use in the veterinary field.
In this paper, the International Lyme and Associated Diseases Society (ILADS) provides its evidence-based definition of chronic Lyme disease. Definition: ILADS defines chronic Lyme disease (CLD) as a multisystem illness with a wide range of symptoms and/or signs that are either continuously or intermittently present for a minimum of six months.
In brief. The use of broad-spectrum antibiotics to treat specific pathogens can damage the host microbiome and contribute to antibiotic resistance. Hygromycin A is selectively taken up through a nucleoside transporter specific to spirochete bacteria, providing a highly selective antibiotic for spirochete infections, such as Lyme disease.
Lyme disease (also known as Lyme borreliosis) is the most common vector-borne disease in the United States with an estimated 476,000 cases per year. ... The research community has largely lacked the necessary funding to properly advance the scientific and clinical understanding of the disease, or to develop and evaluate innovative approaches ...
Lyme disease, which is caused by the spirochete Borrelia burgdorferi, is the most common vectorborne illness in the United States.Although we now know that a form of the illness was recognized in Scandinavia in the early 1900s, modern awareness of Lyme disease began when a cluster of children with "juvenile rheumatoid arthritis" on one small street was reported by their parents in the mid ...
Disease Information. Lyme disease is a multi-system bacterial infection caused by the spirochete, Borrelia burgdorferi, which is transmitted to humans through the bite of an infected deer tick.In early Lyme disease patients can present with a rash and/or flu-like symptoms, but in later stages symptoms can manifest in multiple body systems including musculoskeletal, heart, brain, and nervous ...
Introduction. Lyme disease (LD) is the most common tick-borne infection in North America [1,2].It was first publically recognized in the United States in 1975 in the towns of Lyme and Old Lyme Connecticut as a result of an investigation into 51 cases (39 children) with a similar form of arthritis, although the first case was describe five years earlier by a dermatologist in Wisconsin [3,4].
"Most of the antibiotics we still use are based on discoveries that are decades old, and antibiotic resistance is an increasing problem across many bacterial diseases," says Peter Gwynne, first author on the paper and research assistant professor of molecular biology and microbiology at Tufts University School of Medicine and the Tufts Lyme ...
ABSTRACT. There are increasing levels of concern about policy and practice related to Lyme disease testing and diagnosis in the UK. A complex debate has emerged, with patient groups challenging clinical and testing practices.
Public health warnings about vector-borne diseases in the US are heating up. In a May editorial, scientists from the Centers for Disease Control and Prevention (CDC) made a plea for a vaccine against West Nile virus, which is spread by mosquitoes and has no treatment.By midsummer, the CDC reported that as many as 450 000 people in the US may have a meat allergy from the bite of the lone star ...
The state of the science relating to prolonged symptoms associated with Lyme disease is limited, emerging, and unsettled. Additional research is needed to better understand how to treat, manage, and support people with prolonged symptoms associated with Lyme disease. May 14, 2024 Sources Print Share. Facebook LinkedIn Twitter Syndicate.