Advertisement
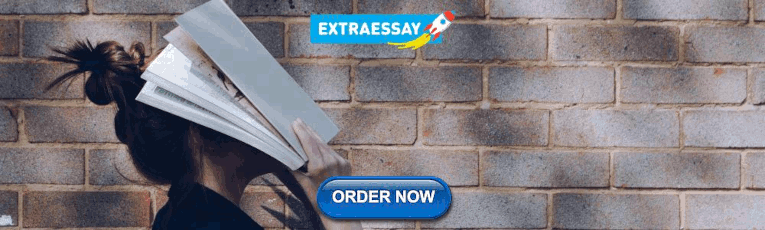
Sustainable agricultural practices for food security and ecosystem services
- Review Article
- Published: 18 October 2022
- Volume 29 , pages 84076–84095, ( 2022 )
Cite this article
- Abdul Rehman 1 ,
- Muhammad Farooq ORCID: orcid.org/0000-0003-4368-9357 3 , 4 ,
- Dong-Jin Lee 2 &
- Kadambot H. M. Siddique 4
2602 Accesses
21 Citations
5 Altmetric
Explore all metrics
The notion of food security is a global phenomenon that impinges on every human. Efforts to increase productivity and yields have historically degraded the environment and reduced biodiversity and ecosystem services, with the significant impact on the poor. Sustainable agriculture—farming in sustainable ways based on an understanding of ecosystem services—is a practical option for achieving global food security while minimizing further environmental degradation. Sustainable agricultural systems offer ecosystem services, such as pollination, biological pest control, regulation of soil and water quality, maintenance of soil structure and fertility, carbon sequestration and mitigation of greenhouse gas emissions, nutrient cycling, hydrological services, and biodiversity conservation. In this review, we discuss the potential of sustainable agriculture for achieving global food security alongside healthy ecosystems that provide other valuable services to humankind. Too often, agricultural production systems are considered separate from other natural ecosystems, and insufficient attention has been paid to how services can flow to and from agricultural production systems to surrounding ecosystems. This review also details the trade-offs and synergies between ecosystem services, highlights current knowledge gaps, and proposes areas for future research.
This is a preview of subscription content, log in via an institution to check access.
Access this article
Price includes VAT (Russian Federation)
Instant access to the full article PDF.
Rent this article via DeepDyve
Institutional subscriptions
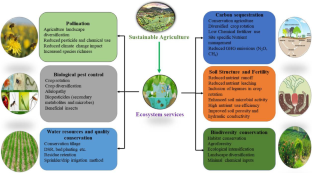
Similar content being viewed by others
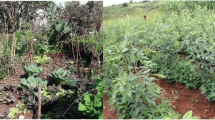
Agroecological principles and elements and their implications for transitioning to sustainable food systems. A review
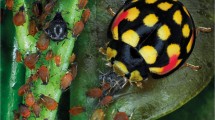
Integrated pest management: good intentions, hard realities. A review
Traditional agriculture: a climate-smart approach for sustainable food production, data availability.
The datasets used and/or analyzed during the current study are available from the corresponding author on reasonable request.
Aguilera E, Lassaletta L, Sanz-Cobena A, Garnier J, Vallejo A (2013) The potential of organic fertilizers and water management to reduce N 2 O emissions in Mediterranean climate ffigcropping systems. A Review Agric Ecosyst Environ 164:32–52
Article CAS Google Scholar
Alam MZ, Crump AR, Haque M, Islam M, Hossain E, Hasan SB, Hasan SB, Hossain M (2016) Effects of integrated pest management on pest damage and yield components in a rice agro-ecosystem in the Barisal Region of Bangladesh. Front Environ Sci 4:22
Article Google Scholar
Alam MK, Salahin N, Islam S, Begum RA, Hasanuzzaman M, Islam MS, Rahman MM (2017) Patterns of change in soil organic matter, physical properties and crop productivity under tillage practices and cropping systems in Bangladesh. J Agri Sci 155:216–238
Albrecht M, Schmid B, Hautier Y, Müller CB (2012) Diverse pollinator communities enhance plant reproductive success. Proc Royal Soc B Biol Sci 279:4845–4852
Bajwa AA, Mahajan G, Chauhan BS (2015) Nonconventional weed management strategies for modern agriculture. Weed Sci 63:723–747
Balbi S, del Prado A, Gallejones P, Geevan CP, Pardo G, Pérez-Miñana E, Manrique R, Hernandez-Santiago C, Villa F (2015) Modelling trade-offs among ecosystem services in agricultural production systems. Environ Modell Softw 72:314–326
Banaszak-Cibicka W, Takacs V, Kesy M, Langowska A, Blecharczyk A, Sawinska Z, Sparks TH, Tryjanowski P (2019) Manure application improves both bumblebee flower visitation and crop yield in intensive farmland. Basic Appl Ecol 36:26–33
Barrett SC (2003) Mating strategies in flowering plants: the outcrossing–selfing paradigm and beyond. Philos Trans R Soc Lond Ser B Biol Sci 358:991–1004
Bedano JC, Domínguez A, Arolfo R, Wall LG (2016) Effect of good agricultural practices under no till on litter and soil invertebrates in areas with different soil types. Soil Tillage Res 158:100–109
Beier RC, Byrd JA, Kubena LF, Hume ME, McReynolds JL, Anderson RC, Nisbet DJ (2014) Evaluation of linalool, a natural antimicrobial and insecticidal essential oil from basil: Effects on poultry. Poult Sci 93:267–272
Bhan S, Behera UK (2014) Conservation agriculture in India–problems, prospects and policy issues. Int Soil Water Conser Res 2:1–12
Bhatt R, Kukal SS, Busari MA, Arora S, Yadav M (2016) Sustainability issues on rice-wheat cropping system. Int Soil Water Conser Res 4:68–83
Bianchi FJ, Booij CJH, Tscharntke T (2006) Sustainable pest regulation in agricultural landscapes: a review on landscape composition, biodiversity and natural pest control. Proc Royal Soc B Biol Sci 273:1715–1727
Blesh J, Hoey L, Jones AD, Friedmann H, Perfecto I (2019) Development pathways toward “zero hunger.” World Dev 118:1–14
Boedecker J, Odhiambo Odour F, Lachat C, Van Damme P, Kennedy G, Termote C (2019) Participatory farm diversification and nutrition education increase dietary diversity in Western Kenya. Matern. Child Nutr 15:e12803
Bommarco R, Marini L, Vaissière BE (2012) Insect pollination enhances seed yield, quality, and market value in oilseed rape. Oecologia 169:1025–1032
Boreux V, Kushalappa CG, Vaast P, Ghazoul J (2013) Interactive effects among ecosystem services and management practices on crop production: pollination in coffee agroforestry systems. Proc Natl Acad Sci USA 110:8387–8392
Bronick CJ, Lal R (2005) Soil structure and management: a review. Geoderma 124:3–22
Burney JA, Davis SJ, Lobell DB (2010) Greenhouse gas mitigation by agricultural intensification. Proc Natl Acad Sci USA 107:12052–12057
Campbell CA, Janzen HH, Paustian K, Gregorich EG, Sherrod L, Liang BC, Zentner RP (2005) Carbon storage in soils of the North American Great Plains: effect of cropping frequency. Agron J 97:349–363
Campos EV, Proença PL, Oliveira JL, Bakshi M, Abhilash PC, Fraceto LF (2019) Use of botanical insecticides for sustainable agriculture: future perspectives. Ecol Indicat 105:483–495
Cardinale BJ, Duffy JE, Gonzalez A, Hooper DU, Perrings C, Venail P, Narwani A, Mace GM, Tilman D, Wardle DA, Kinzig AP (2012) Biodiversity loss and its impact on humanity. Nature 486:59–67
Carretta L, Tarolli P, Cardinali A, Nasta P, Romano N, Masin R (2021) Evaluation of runoff and soil erosion under conventional tillage and no-till management: a case study in northeast Italy. CATENA 197:104972
Carvalheiro LG, Seymour CL, Veldtman R, Nicolson SW (2010) Pollination services decline with distance from natural habitat even in biodiversity-rich areas. J Appl Ecol 47:810–820
Cassman KG, Grassini P (2020) A global perspective on sustainable intensification research. Nature Sustainability 3:262–268
Cassman KG, Dobermann A, Walters DT, Yang H (2003) Meeting cereal demand while protecting natural resources and improving environmental quality. Annu Rev Environ Resour 28:315–358
Cheema ZA, Khaliq A, Tariq M (2002) Evaluation of concentrated sorgaab alone and in combination with reduced rates of three pre-emergence herbicides for weed control in cotton ( Gossypium hirsutum L.). Int J Agric Biol 4:549–552
CAS Google Scholar
Choudhary M, Datta A, Jat HS, Yadav AK, Gathala MK, Sapkota TB, Das AK, Sharma PC, Jat ML, Singh R, Ladha JK (2018) Changes in soil biology under conservation agriculture based sustainable intensification of cereal systems in Indo-Gangetic Plains. Geoderma 313:193–204
Choudhary M, Jat HS, Datta A, Yadav AK, Sapkota TB, Mondal S, Meena RP, Sharma PC, Jat ML (2018) Sustainable intensification influences soil quality, biota, and productivity in cereal-based agroecosystems. Appl Soil Ecol 126:189–198
Cong RG, Smith HG, Olsson O, Brady M (2014) Managing ecosystem services for agriculture: will landscape-scale management pay? Ecol Econ 99:53–62
Google Scholar
Culman SW, Snapp SS, Green JM, Gentry LE (2013) Short-and long-term labile soil carbon and nitrogen dynamics reflect management and predict corn agronomic performance. Agron J 105:493–502
Cycoń M, Mrozik A, Piotrowska-Seget Z (2017) Bioaugmentation as a strategy for the remediation of pesticide-polluted soil: a review. Chemosphere 172:52–71
Daily GC (1997) Nature’s Services, vol 3. Island Press, Washington, DC
Das TK, Saharawat YS, Bhattacharyya R, Sudhishri S, Bandyopadhyay KK, Sharma AR, Jat ML (2018) Conservation agriculture effects on crop and water productivity, profitability and soil organic carbon accumulation under a maize-wheat cropping system in the North-western Indo-Gangetic Plains. Field Crops Res 215:222–231
Dayan FE, Cantrell CL, Duke SO (2009) Natural products in crop protection. Bioorg Med Chem 17:4022–4034
DeFries R, Mondal P, Singh D, Agrawal I, Fanzo J, Remans R, Wood S (2016) Synergies and trade-offs for sustainable agriculture: nutritional yields and climate-resilience for cereal crops in Central India. Global Food Sec 11:44–53
Devendra C, Thomas D (2002) Crop–animal interactions in mixed farming systems in Asia. Agric Syst 71:27–40
Diniz FH, Hoogstra-Klein MA, Kok K, Arts B (2013) Livelihood strategies in settlement projects in the Brazilian Amazon: determining drivers and factors within the Agrarian Reform Program. J Rural Stud 32:196–207
Dinnes DL, Karlen DL, Jaynes DB, Kaspar TC, Hatfield JL, Colvin TS, Cambardella CA (2002) Nitrogen management strategies to reduce nitrate leaching in tile-drained Midwestern soils. Agron J 94:153–171
Du X, Jian J, Du C, Stewart RD (2022) Conservation management decreases surface runoff and soil erosion. International Soil and Water Conservation Research 10(2):188–196
Ebabu K, Tsunekawa A, Haregeweyn N, Adgo E, Meshesha DT, Aklog D, Masunaga T, Tsubo M, Sultan D, Fenta AA, Yibeltal M (2020) Exploring the variability of soil properties as influenced by land use and management practices: a case study in the Upper Blue Nile basin. Ethiopia Soil Tillage Res 200:104614
Engel JB, Heckler C, Tondo EC, Daroit DJ, da Silva MP (2017) Antimicrobial activity of free and liposome-encapsulated thymol and carvacrol against Salmonella and Staphylococcus aureus adhered to stainless steel. Int J Food Microbiol 252:18–23
Evenson RE, Gollin D (2003) Assessing the impact of the Green Revolution, 1960 to 2000. Science 300:758–762
Eze S, Dougill AJ, Banwart SA, Hermans TD, Ligowe IS, Thierfelder C (2020) Impacts of conservation agriculture on soil structure and hydraulic properties of Malawian agricultural systems. Soil Tillage Res 201:104639
Farooq M, Jabran K, Cheema ZA, Wahid A, Siddique KHM (2011) The role of allelopathy in agricultural pest management. Pest Manage Sci 67:493–506
Farooq M, Bajwa AA, Cheema SA, Cheema ZA (2013) Application of allelopathy in crop production. Int J Agric Biol 15:1367–1378
Farooq M, Rehman A, Pisante M (2019) Sustainable agriculture and food security. In Farooq M, Pinsante M (eds). Innovations in Sustainable Agriculture. Springer Nature, Switzerland, pp 3–24
Ferranti P (2016) Food production and ecosystem protection. In: reference module in food science. Elsevier, Amsterdam, The Netherlands. 1–3. https://doi.org/10.1016/B978-0-08-100596-5.03444-2
Foley JA, DeFries R, Asner GP, Barford C, Bonan G, Carpenter SR, Chapin FS, Coe MT, Daily GC, Gibbs HK, Helkowski JH (2005) Global consequences of land use. Science 309:570–574
Food Agriculture Organization (FAO) (2017) The future of food and agriculture – trends and challenges. Annual report. Food and Agriculture Organization of the United Nations, Rome, Italy. 296:1–80
FAO, IFAD, UNICEF, WFP and WHO 2018 The state of food security and nutrition in the world 2018. Building climate resilience for food security and nutrition. Rome, Italy.
Fu X, Wang J, Sainju UM, Liu W (2019) Soil nitrogen fractions under long-term crop rotations in the Loess Plateau of China. Soil Tillage Res 186:42–51
Garbach K, Milder JC, DeClerck FA, Montenegro de Wit M, Driscoll L, Gemmill-Herren B (2017) Examining multi-functionality for crop yield and ecosystem services in five systems of agroecological intensification. Int J Agric Sustain 15:11–28
Gelaw AM, Singh BR, Lal R (2014) Soil organic carbon and total nitrogen stocks under different land uses in a semi-arid watershed in Tigray, Northern Ethiopia. Agric Ecosyst Environ 188:256–263
Gill RJ, Baldock KC, Brown MJ, Cresswell JE, Dicks LV, Fountain MT, Garratt MP, Gough LA, Heard MS, Holland JM, Ollerton J (2016) Protecting an ecosystem service: approaches to understanding and mitigating threats to wild insect pollinators. Adv Ecol Res 54:135–206
Godfray HCJ, Garnett T (2014) Food security and sustainable intensification. Phil Trans R Soc B 369:20120273
Godfray HCJ, Blacquiere T, Field LM, Hails RS, Petrokofsky G, Potts SG, Raine NE, Vanbergen AJ, McLean AR (2014) A restatement of the natural science evidence base concerning neonicotinoid insecticides and insect pollinators. Phil Trans R Soc B 281:20140558
Gómez MI, Barrett CB, Raney T, Pinstrup-Andersen P, Meerman J, Croppenstedt A, Carisma B, Thompson B (2013) Post-green revolution food systems and the triple burden of malnutrition. Food Policy 42:129–138
Gómez-Baggethun E, De Groot R, Lomas PL, Montes C (2010) The history of ecosystem services in economic theory and practice: from early notions to markets and payment schemes. Ecol Econ 69:1209–1218
Gomiero T, Pimentel D, Paoletti MG (2011) Is there a need for a more sustainable agriculture? Crit Rev Plant Sci 30:6–23
Gonzalez JM (2018) Runoff and losses of nutrients and herbicides under long-term conservation practices (no-till and crop rotation) in the US Midwest: a variable intensity simulated rainfall approach. International Soil and Water Conservation Research 6(4):265–274
Gonzalez-Sanchez EJ, Veroz-Gonzalez O, Conway G, Moreno-Garcia M, Kassam A, Mkomwa S, Ordoñez-Fernandez R, Triviño-Tarradas P, Carbonell-Bojollo R (2019) Meta-analysis on carbon sequestration through Conservation Agriculture in Africa. Soil Tillage Res 190:22–30
Goshu D, Kassa B, Ketema M (2013) Is food security enhanced by agricultural technologies in rural Ethiopia? Afr J Agric Res Econ 8:58–68
Grassini P, Eskridge KM, Cassman KG (2013) Distinguishing between yield advances and yield plateaus in historical crop production trends. Nat Commun 4:1–11
Gurr GM, Lu Z, Zheng X, Xu H, Zhu P, Chen G, Yao X, Cheng J, Zhu Z, Catindig JL, Villareal S (2016) Multi-country evidence that crop diversification promotes ecological intensification of agriculture. Nat Plants 2:22–25
De Haan C, Steinfield H, Blackburn H (1997) Livestock and the environment. Finding a balance European Commission Directorate-General for Development, Development Policy Sustainable Development and Natural Resources. Rome, Italy, p 115
Hao X, He W, Lam SK, Li P, Zong Y, Zhang D, Li FY (2020) Enhancement of no-tillage, crop straw return and manure application on field organic matter content overweigh the adverse effects of climate change in the arid and semi-arid Northwest China. Agric for Meteorol 295:108199
Harb OM, Abd El-Hay GH, Hager MA, Abou El-Enin MM (2015) Studies on conservation agriculture in Egypt. Ann Agric Sci 60:105–112
Hendrickson O (2003) Influences of global change on carbon sequestration by agricultural and forest soils. Environ Rev 11:161–192
Herman A, Tambor K, Herman A (2016) Linalool affects the antimicrobial efficacy of essential oils. Curr Microbiol 72:165–172
Huang J, Yang G (2017) Understanding recent challenges and new food policy in China. Global Food Sec 12:119–126
Huang Y, Ren W, Wang L, Hui D, Grove JH, Yang X, Tao B, Goff B (2018) Greenhouse gas emissions and crop yield in no-tillage systems: a meta-analysis. Agric Ecosyst Environ 268:144–153
IPBES (2016) The assessment report of the Intergovernmental Science‐Policy Platform on Biodiversity and Ecosystem Services on pollinators, pollination and food production. In Potts SG, Imperatriz‐Fonseca VL, Ngo HT (eds), Secretariat of the Intergovernmental Science‐Policy Platform on Biodiversity and Ecosystem Services, Bonn, Germany, p 827
Islam S, Gathala MK, Tiwari TP, Timsina J, Laing AM, Maharjan S, Chowdhury AK, Bhattacharya PM, Dhar T, Mitra B, Kumar S (2019) Conservation agriculture based sustainable intensification: increasing yields and water productivity for smallholders of the Eastern Gangetic Plains. Field Crops Res 238:1–17
Jabran K, Cheema ZA, Farooq M, Hussain M (2010) Lower doses of pendimethalin mixed with allelopathic crop water extracts for weed management in canola ( Brassica napus ). Int J Agric Biol 12:335–340
Jabran K, Mahajan G, Sardana V, Chauhan BS (2015) Allelopathy for weed control in agricultural systems. Crop Protec 72:57–65
Jarvis NJ (2017) A review of non-equilibrium water flow and solute transport in soil macropores: principles, controlling factors and consequences for water quality. Eur J Soil Sci 58:523–546
Jat RK, Singh RG, Kumar M, Jat ML, Parihar CM, Bijarniya D, Sutaliya JM, Jat MK, Parihar MD, Kakraliya SK, Gupta RK (2019) Ten years of conservation agriculture in a rice–maize rotation of Eastern Gangetic Plains of India: yield trends, water productivity and economic profitability. Field Crops Res 232:1–10
Jat SL, Parihar CM, Singh AK, Nayak HS, Meena BR, Kumar B, Parihar MD, Jat ML (2019) Differential response from nitrogen sources with and without residue management under conservation agriculture on crop yields, water-use and economics in maize-based rotations. Field Crops Res 236:96–110
Jiang J, Guo S, Zhang Y, Liu Q, Wang R, Wang Z, Li N, Li R (2015) Changes in temperature sensitivity of soil respiration in the phases of a three-year crop rotation system. Soil Tillage Res 150:139–146
Kandhro MN, Tunio S, Rajpar I, Chachar Q (2014) Allelopathic impact of sorghum and sunflower intercropping on weed management and yield enhancement in cotton. Sarhad J Agric Sci 30:311–318
Kansanga MM, Kangmennaang J, Kerr RB, Lupafya E, Dakishoni L, Luginaah I (2021) Agroecology and household production diversity and dietary diversity: evidence from a five-year agroecological intervention in rural Malawi. Soc Sci Med 288:113550. https://doi.org/10.1016/j.socscimed.2020.113550
Kearns CA, Inouye DW, Waser NM (1998) Endangered mutualisms: the conservation of plant-pollinator interactions. Annu Rev Ecol System 29:83–112
Kennedy CM, Lonsdorf E, Neel MC, Williams NM, Ricketts TH, Winfree R, Bommarco R, Brittain C, Burley AL, Cariveau D, Carvalheiro LG (2013) A global quantitative synthesis of local and landscape effects on wild bee pollinators in agroecosystems. Ecol Lett 16:584–599
Kerr JT, Pindar A, Galpern P, Packer L, Potts SG, Roberts SM, Rasmont P, Schweiger O, Colla SR, Richardson LL, Wagner DL (2015) Climate change impacts on bumblebees converge across continents. Science 349:177–180
Kleijn D, Bommarco R, Fijen TP, Garibaldi LA, Potts SG, van der Putten WH (2019) Ecological intensification: bridging the gap between science and practice. Trend Ecol Evol 34:154–166
Klein AM, Vaissiere BE, Cane JH, Steffan-Dewenter I, Cunningham SA, Kremen C, Tscharntke T (2007) Importance of pollinators in changing landscapes for world crops. Proc Royal Soc B Biol Sci 274:303–313
Konikow LF, Kendy E (2005) Groundwater depletion: a global problem. Hydrogeol J 13:317–320
Kragt ME, Robertson MJ (2014) Quantifying ecosystem services trade-offs from agricultural practices. Ecol Econ 1(102):147–157
Kremen C, Miles A (2012) Ecosystem services in biologically diversified versus conventional farming systems: benefits, externalities, and trade-offs. Ecol Soc 17:40
Krupinsky JM, Tanaka DL, Merrill SD, Liebig MA, Hanson JD (2006) Crop sequence effects of 10 crops in the northern Great Plains. Agric Syst 88:227–254
Kumar S, Singh A (2014) Biopesticides for integrated crop management: environmental and regulatory aspects. J. Biofertil Biopestici 5–e121
Kumar N, Nath CP (2019) Impact of zero-till residue management and crop diversification with legumes on soil aggregation and carbon sequestration. Soil Till Res 189:158–167
Lal R (2001) Soil degradation by erosion. Land Degrad Develop 12:519–539
Lal R (2004) Soil carbon sequestration to mitigate climate change. Geoderma 123:1–22
Lal R (2008) Carbon sequestration. Philosop. Transac Royal Soc b: Biol Sci 363:815–830
Lal R, Follett RF, Stewart BA, Kimble JM (2007) Soil carbon sequestration to mitigate climate change and advance food security. Soil Sci 172:943–956
Landis DA (2017) Designing agricultural landscapes for biodiversity-based ecosystem services. Basic Appl Ecol 18:1–12
Langeroodi ARS, Osipitan OA, Radicetti E (2019) Benefits of sustainable management practices on mitigating greenhouse gas emissions in soybean crop ( Glycine max ). Sci Total Environ 660:1593–1601
Lechenet M, Bretagnolle V, Bockstaller C, Boissinot F, Petit MS, Petit S, Munier-Jolain NM (2014) Reconciling pesticide reduction with economic and environmental sustainability in arable farming. PLoS ONE 9:e97922
Lemke AM, Kirkham KG, Lindenbaum TT, Herbert ME, Tear TH, Perry WL, Herkert JR (2011) Evaluating agricultural best management practices in tile-drained subwatersheds of the Mackinaw River, Illinois. J Environ Qual 40:1215–1228
Liu L, Greaver TL (2009) A review of nitrogen enrichment effects on three biogenic GHGs: the CO 2 sink may be largely offset by stimulated N 2 O and CH 4 emission. Ecol Lett 12:1103–1117
Lovo S (2016) Tenure insecurity and investment in soil conservation. Evidence from Malawi. World Develop 78:219–229
Mabhaudhi T et al (2019) Mainstreaming underutilized indigenous and traditional crops into food systems: a South African perspective. Sustainability 11:172
Macias FA, Molinillo JM, Varela RM, Galindo JC (2007) Allelopathy—a natural alternative for weed control. Pest Manag Sci 63:327–348
Mäder P, Fliessbach A, Dubois D, Gunst L, Fried P, Niggli U (2002) Soil fertility and biodiversity in organic farming. Science 296:1694–1697
Majumder B, Mandal B, Bandyopadhyay PK, Gangopadhyay A, Mani PK, Kundu AL, Mazumdar D (2008) Organic amendments influence soil organic carbon pools and rice wheat productivity. Soil Sci Soc Am J 72:775–785
Mensah AK (2015) Role of revegetation in restoring fertility of degraded mined soils in Ghana: a review. Int J Biodivers Conserv 7:57–80
Mirzaei M, Anari MG, Razavy-Toosi E, Zaman M, Saronjic N, Zamir SM, Mohammed S, Caballero-Calvo A (2022) Crop residues in corn-wheat rotation in a semi-arid region increase CO2 efflux under conventional tillage but not in a no-tillage system. Pedobiologia 93:150819
Mishra B, Gyawali BR, Paudel KP, Poudyal NC, Simon MF, Dasgupta S, Antonious G (2018) Adoption of sustainable agriculture practices among farmers in Kentucky, USA. Environ Manag 62:1060–1072
Mloza-Banda ML, Cornelis WM, Mloza-Banda HR, Makwiza CN, Verbist K (2014) Soil properties after change to conservation agriculture from ridge tillage in sandy clay loams of mid-altitude Central Malawi. Soil Use Manag 30:569–578
Mloza-Banda HR, Makwiza CN, Mloza-Banda ML (2016) Soil properties after conversion to conservation agriculture from ridge tillage in Southern Malawi. J Arid Environ 127:7–16
Morales CL, Arbetman MP, Cameron SA, Aizen MA (2013) Rapid ecological replacement of a native bumble bee by invasive species. Front Ecol Environ 11:529–534
Nawaz A, Farooq M, Lal R, Rehman A, Hussain T, Nadeem A (2017) Influence of sesbania brown manuring and rice residue mulch on soil health, weeds and system productivity of conservation rice–wheat systems. Land Degrad Develop 28:1078–1090
Nawaz A, Lal R, Shrestha RK, Farooq M (2017) Mulching affects soil properties and greenhouse gas emissions under long-term no-till and plough-till systems in Alfisol of central Ohio. Land Degrad Develop 28:673–681
Naylor R, Ehrlich, P (1997) Natural pest control services and agriculture. In: Daily, G. (ed). Nature's services: Societal dependence on natural ecosystems. Island Press, Washington, 151–174
Newbold T, Hudson LN, Hill SL, Contu S, Lysenko I, Senior RA, Börger L, Bennett DJ, Choimes A, Collen B, Day J (2015) Global effects of land use on local terrestrial biodiversity. Nature 520:45–50
Nicholson CC, Koh I, Richardson LL, Beauchemin A, Ricketts TH (2017) Farm and landscape factors interact to affect the supply of pollination services Agric Ecosyst Environ 250:113–122
Omonode RA, Vyn TJ, Smith DR, Hegymegi P, Gál A (2007) Soil carbon dioxide and methane fluxes from long-term tillage systems in continuous corn and corn–soybean rotations. Soil till Res 95:182–195
Pachauri RK, Allen MR, Barros VR, Broome J, Cramer W, Christ R, Church JA, Clarke L, Dahe Q, Dasgupta P, Dubash NK, Edenhofer O, Elgizouli I, Field CB, Forster P, Friedlingstein P, Fuglestvedt J, Gomez-Echeverri L, Hallegatte S, Hegerl G, Howden M, Jiang K, Jimenez Cisneroz B, Kattsov V, Lee H, Mach KJ, Marotzke J, Mastrandrea MD, Meyer L, Minx J, Mulugetta Y, O'Brien K, Oppenheimer M, Pereira JJ, Pichs-Madruga R, Plattner GK, Pörtner HO, Power SB, Preston B, Ravindranath NH, Reisinger A, Riahi K, Rusticucci M, Scholes R, Seyboth K, Sokona Y, Stavins R, Stocker TF, Tschakert P, van Vuuren D, van Ypserle JP (2014) Climate Change 2014: synthesis report. Contribution of working groups I, II and III to the Fffth assessment report of the intergovernmental panel on climate change. In: Pachauri R, Meyer L (eds). , Geneva, Switzerland, IPCC, p 151
Parihar CM, Parihar MD, Sapkota TB, Nanwal RK, Singh AK, Jat SL, Nayak HS, Mahala DM, Singh LK, Kakraliya SK, Stirling CM (2018) Long-term impact of conservation agriculture and diversified maize rotations on carbon pools and stocks, mineral nitrogen fractions and nitrous oxide fluxes in inceptisol of India. Sci Total Environ 640:1382–1392
Pascual U, Termansen M, Hedlund K, Brussaard L, Faber JH, Foudi S, Lemanceau P, Jørgensen SL (2015) On the value of soil biodiversity and ecossystem services. Ecosyst Serv 15:1–18
Pascual U, Balvanera P, Díaz S, Pataki G, Roth E, Stenseke M, Watson RT, Dessane EB, Islar M, Kelemen E, Maris V (2017) Valuing nature’s contributions to people: the IPBES approach. Curr Opin Environ Sust 26:7–16
Pavela R, Benelli G (2016) Essential oils as ecofriendly biopesticides? Challenges and constraints. Trend Plant Sci 21:1000–1007
Peng Z, Wang L, Xie J, Li L, Coulter JA, Zhang R, Luo Z, Cai L, Carberry P, Whitbread A (2020) Conservation tillage increases yield and precipitation use efficiency of wheat on the semi-arid Loess Plateau of China. Agric Water Manag 231:106024
Pereira P, Bogunovic I, Muñoz-Rojas M, Brevik EC (2018) Soil ecosystem services, sustainability, valuation and management. Curr Opin Environ Sci Health 5:7–13
Poeplau C, Don A (2015) Carbon sequestration in agricultural soils via cultivation of cover crops–a meta-analysis. Agric Ecosyst Environ 200:33–41
Ponisio LC, M’Gonigle LK, Mace KC, Palomino J, De Valpine P, Kremen C (2015) Diversification practices reduce organic to conventional yield gap. Proc Royal Soc London 282:20141396
Poore J, Nemecek T (2018) Reducing food’s environmental impacts through producers and consumers. Science 360:987–992
Pratibha G, Srinivas I, Rao KV, Shanker AK, Raju BMK, Choudhary DK, Rao KS, Srinivasarao C, Maheswari M (2016) Net global warming potential and greenhouse gas intensity of conventional and conservation agriculture system in rainfed semi-arid tropics of India. Atmos Environ 145:239–250
Pretty J (1997) The sustainable intensification of agriculture. Nat Resour Forum 21:247–256
Pretty J, Bharucha ZP (2014) Sustainable intensifcation in agricultural systems. Ann Bot 114:1571–1596
Pretty J et al (2018) Global assessment of agricultural system redesign for sustainable intensification. Nat Sustain 1:441–446
Puigdefábregas J (2005) The role of vegetation patterns in structuring runoff and sediment fluxes in drylands. Earth Surface Process Landform 30:133–147
Qi X, Fu Y, Wang RY, Ng CN, Dang H, He Y (2018) Improving the sustainability of agricultural land use: an integrated framework for the conflict between food security and environmental deterioration. Appl Geogr 90:214–223
Rader R, Bartomeus I, Garibaldi LA, Garratt MP, Howlett BG, Winfree R, Cunningham SA, Mayfield MM, Arthur AD, Andersson GK, Bommarco R (2016) Non-bee insects are important contributors to global crop pollination. Proc Natl Acad Sci USA 113:146–151
Raudsepp-Hearne C, Peterson GD, Tengö M, Bennett EM, Holland T, Benessaiah K, MacDonald GK, Pfeifer L (2010) Untangling the environmentalists paradox: why is human well-being increasing as ecosystem services degrade? Bioscience 60:576–589
Regnault-Roger C, Vincent C, Arnason JT (2012) Essential oils in insect control: lowrisk products in a high-stakes world. Ann Rev Entomol 57:405–424
Rehman A, Farooq M, Ullah A, Nadeem F, Im SY, Park SK, Lee DJ (2020) Agronomic biofortification of zinc in Pakistan: status, benefits, and constraints. Frontiers in sustainable food systems 4: 591722
Reid WV, Mooney HA, Cropper A, Capistrano D, Carpenter SR, Chopra K, Dasgupta P, Dietz T, Duraiappah AK, Hassan R, Kasperson R (2005) Ecosystems and human well-being-synthesis: a report of the Millennium Ecosystem Assessment. Island Press
Rhodes CJ (2016) The 2015 Paris climate change conference: COP21. Sci Prog 99:97–104
Ryffel AN, Rid W, Grêt-Regamey A (2014) Land use trade-offs for flood protection: a choice experiment with visualizations. Ecosyst Serv 10:111–123
Saharawat YS, Ladha JK, Pathak H, Gathala MK, Chaudhary N, Jat ML (2012) Simulation of resource-conserving technologies on productivity, income and greenhouse gas GHG emission in rice-wheat system. J Soil Sci Environ Manage 3:9–22
Samal SK, Rao KK, Poonia SP, Kumar R, Mishra JS, Prakash V, Mondal S, Dwivedi SK, Bhatt BP, Naik SK, Choubey AK (2017) Evaluation of long-term conservation agriculture and crop intensification in rice-wheat rotation of Indo-Gangetic Plains of South Asia: Carbon dynamics and productivity. Eur J Agron 90:198–208
Sánchez-Navarro V, Zornoza R, Faz Á, Fernández JA (2019) Does the use of cowpea in rotation with a vegetable crop improve soil quality and crop yield and quality? A field study in SE Spain. Eur J Agron 107:10–17
Sánchez-Navarro V, Zornoza R, Faz Á, Fernández JA (2019) Comparing legumes for use in multiple cropping to enhance soil organic carbon, soil fertility, aggregates stability and vegetables yields under semi-arid conditions. Sci Hort 246:835–841
Sapkota TB (2019) Cost-effective opportunities for climate change mitigation in Indian agriculture. Sci Total Environ 655:1342–1354
Sarker KK, Sarkar PK, Hoque MA, Malaker PK, Islam S (2009) J Agric Eng Inst Eng Bangladesh 37:112–118
Sayed A, Sarker A, Kim JE, Rahman M, Mahmud MGA (2020) Environmental sustainability and water productivity on conservation tillage of irrigated maize in red brown terrace soil of Bangladesh. J Saud Soc Agric Sci 19:276–284
Scherer LA, Verburg PH, Schulp CJ (2018) Opportunities for sustainable intensification in European agriculture. Global Environ Change 48:43–55
Seufert V, Ramankutty N, Foley JA (2012) Comparing the yields of organic and conventional agriculture. Nature 485:229–232
Shahzad M, Farooq M, Jabran K, Hussain M (2016) Impact of different crop rotations and tillage systems on weed infestation and productivity of bread wheat. Crop Protec 89:161–169
Sidhu HS, Jat ML, Singh Y, Sidhu RK, Gupta N, Singh P, Singh P, Jat HS, Gerard B (2019) Sub-surface drip fertigation with conservation agriculture in a rice-wheat system: a breakthrough for addressing water and nitrogen use efficiency. Agric Water Manag 216:273–283
Silva JV, Reidsma P, Baudron F, Laborte AG, Giller KE, van Ittersum MK (2021) How sustainable is sustainable intensification? assessing yield gaps at field and farm level across the globe. Glob Food Sec 30:100552
Six J, Ogle SM, Jay Breidt F, Conant RT, Mosier AR, Paustian K (2004) The potential to mitigate global warming with no-tillage management is only realized when practiced in the long term. Glob Chang Biol 10:155–160
Smith P, Gregory PJ (2013) Climate change and sustainable food production. Proc Nutr Soc 72:21–28
Smith P, Martino D, Cai Z, Gwary D, Janzen H, Kumar P, McCarl B, Ogle S, O’Mara F, Rice C, Scholes B (2008) Greenhouse gas mitigation in agriculture. Philos. Trans. R. Soc Lond Ser B Biol Sci 363:789–813
Smith P, Martino D, Cai Z, Gwary D, Janzen H, Kumar P, McCarl B, Ogle S, O’Mara F, Rice C, Scholes B, Sirotenko O (2007) Agriculture. In: Metz B, Davidson OR, Bosch PR, Dave R, Meyer A (eds) Climate Change 2007: Mitigation. Contribution of Working Group III to the Fourth Assessment Report of the Intergovernmental Panel on Climate Change. Cambridge University Press, Cambridge, United Kingdom and New York, NY, USA, pp 497–540
Snapp SS, Grabowski P, Chikowo R, Smith A, Anders E, Sirrine D, Chimonyo V, Bekunda M (2018) Maize yield and profitability tradeoffs with social, human and environmental performance: is sustainable intensification feasible? Agric Syst 162:77–88
Stagnari F, Ramazzotti S, Pisante M (2009) Conservation agriculture: a different approach for crop production through sustainable soil and water management: a review. In: Lichtfouse E (ed) Organic Farming, Pest Control and Remediation Of Soil Pollutants. Springer, Dordrecht, pp 55–83
Chapter Google Scholar
Stagnari F, Jan S, Galieni A, Pisante M (2016) Sustainable agricultural practices for water quality protection. In: Ahmad, P. (ed.). Water stress and crop plants: A sustainable Approach. John Wiley & Sons, Ltd. Chichester, UK, 75–85
IPCC (2013) Climate change 2013: the physical science basis. In: Stocker TF, Qin D, Plattner GK, Tignor MM, Allen SK, Boschung J, Nauels A, Xia Y, Bex V, Midgley PM (eds.). Contribution of working group I to the fifth assessment report of the Intergovernmental panel on climate change. Cambridge University Press, Cambridge, United Kingdom and New York, NY, USA, 1535
Subbulakshmi S, Harisudan C, Saravanan N, Subbian P (2009) Conservation tillage–an ecofriendly management practices for agriculture. Res J Agric Biol Sci 5:1098–1110
Swinton SM, Lupi F, Robertson GP, Landis DA (2006) Ecosystem services from agriculture: looking beyond the usual suspects. Am J Agric Econ 88:1160–1166
Tellez-Rio A, Vallejo A, Garcia-Marco S, Martin-Lammerding D, Tenorio JL, Rees RM, Guardia G (2017) Conservation Agriculture practices reduce the global warming potential of rainfed low N input semi-arid agriculture. Eur J Agron 84:95–104
Thomson AM, Ellis EC, Grau HR, Kuemmerle T, Meyfroidt P, Ramankutty N, Zeleke G (2019) Sustainable intensification in land systems: trade-offs, scales, and contexts. Curr Opin Environ Sustain 38:37–43
Tilman D (1999) Global environmental impacts of agricultural expansion: the need for sustainable and efficient practices. Proc Natl Acad Sci 96:5995–6000
Tilman D, Balzer C, Hill J, Befort BL (2011) Global food demand and the sustainable intensification of agriculture. Proc Natl Acad Sci 108:20260–20264
Tirol-Padre A, Rai M, Kumar V, Gathala M, Sharma PC, Sharma S, Nagar RK, Deshwal S, Singh LK, Jat HS, Sharma DK (2016) Quantifying changes to the global warming potential of rice wheat systems with the adoption of conservation agriculture in northwestern India. Agric Ecosyst Environ 219:125–213
Tittonell P, Giller KE (2013) When yield gaps are poverty traps: the paradigm of ecological intensification in African smallholder agriculture. Field Crops Res 143:76–90
Tscharntke T, Klein AM, Kruess A, Steffan-Dewenter I, Thies C (2005) Landscape perspectives on agricultural intensification and biodiversity–ecosystem service management. Ecol Lett 8:857–874
Tscharntke T, Clough Y, Wanger TC, Jackson L, Motzke I, Perfecto I, Vandermeer J, Whitbread A (2012) Global food security, biodiversity conservation and the future of agricultural intensification. Biol Conserv 151:53–59
Tubiello FN, Soussana JF, Howden SM (2007) Crop and pasture response to climate change. Proc Natl Acad Sci 104:19686–19690
Tubiello FN, Salvatore M, Cóndor Golec RD, Ferrara A, Rossi S, Biancalani R, Federici S, Jacobs H, Flammini A (2014) Agriculture, forestry and other land use emissions by sources and removals by sinks. Statistics Division, Food and Agriculture Organization, Rome.
Turpin N, Ten Berge H, Grignani C, Guzmán G, Vanderlinden K, Steinmann HH, Siebielec G, Spiegel A, Perret E, Ruysschaert G, Laguna A (2017) An assessment of policies affecting Sustainable Soil Management in Europe and selected member states. Land Use Policy 66:241–249
Van Zanten BT, Verburg PH, Espinosa M, Gomez-y-Paloma S, Galimberti G, Kantelhardt J, Kapfer M, Lefebvre M, Manrique R, Piorr A, Raggi M (2014) European agricultural landscapes, comon agricultural policy and ecosystem services: a review. Agron Sustain Dev 34:309–325
Verburg K, Bond WJ, Hunt JR (2012) Fallow management in dryland agriculture: explaining soil water accumulation using a pulse paradigm. Field Crop Res 130:68–79
Verger PJ, Boobis AR (2013) Reevaluate pesticides for food security and safety. Science 341:717–718
Vincent JR (2012) Ecosystem services and green growth (The World Bank 2012). Online available at https://openknowledge.worldbank.org/bitstream/handle/10986/12084/wps6233.pdf?sequence=1&isAllowed=y Accessed on 7 July 10, 2020
Wagstaff P, Harty M (2010) The impact of conservation agriculture on food security in three low veldt districts of Zimbabwe. Trocaire Develop Rev 2010:67–84
Waha K, Van Wijk MT, Fritz S, See L, Thornton PK, Wichern J, Herrero M (2018) Agricultural diversification as an important strategy for achieving food security in Africa. Glob. Change Biol 24:3390–400
Weiss CM, Fox K (2003) European food supply chains – are they sustainable? Execut. Outlook 3:54–63
Wossink A, Swinton SM (2007) Jointness in production and farmers’ willingness to supply non-marketed ecosystem services. Ecol Econ 64:297–304
Xie Z, Tu S, Shah F, Xu C, Chen J, Han D, Liu G, Li H, Muhammad I, Cao W (2016) Substitution of fertilizer-N by green manure improves the sustainability of yield in double-rice cropping system in south China. Field Crops Res 188:142–149
Xuan TD, Shinkichi T, Khanh TD, Min CI (2005) Biological control of weeds and plant pathogens in paddy rice by exploiting plant allelopathy: an overview. Crop Prot 24:197–206
Zhang W, Ricketts TH, Kremen C, Carney K, Swinton SM (2007) Ecosystem services and dis-services to agriculture. Ecol Econ 64:253–260
Zhang ZS, Chen J, Liu TQ, Cao CG, Li CF (2016) Effects of nitrogen fertilizer sources and tillage practices on greenhouse gas emissions in paddy fields of central China. Atmos Environ 144:274–281
Download references
This study received financial support from the “His Majesty Trust Fund” Sultan Qaboos University (SR/AGR/CROP/19/01).
Author information
Authors and affiliations.
Department of Agronomy, Faculty of Agriculture and Environment, The Islamia University of Bahawalpur, Bahawalpur, 63100, Pakistan
Abdul Rehman
Department of Crop Sciences and Biotechnology, Dankook University, Cheonan-si, 31116, South Korea
Dong-Jin Lee
Department of Plant Sciences, College of Agricultural and Marine Sciences, Sultan Qaboos University, Al-Khoud 123, Muscat, Oman
Muhammad Farooq
The UWA Institute of Agriculture, The University of Western Australia, Perth, WA, 6001, Australia
Muhammad Farooq & Kadambot H. M. Siddique
You can also search for this author in PubMed Google Scholar
Contributions
AR collected the literature, prepared the first draft, and revised the manuscript; MF conceptualized the idea, edited and improved the manuscript; DJL edited and improved the manuscript; KHM edited and improved the manuscript.
Corresponding author
Correspondence to Muhammad Farooq .
Ethics declarations
Ethical approval and consent to participate.
This study did not involve human participants, human data, or human tissue.
Consent to publish
All authors agreed to publish the article.
Conflict of interest
The authors declare no competing interests.
Additional information
Responsible Editor: Philippe Garrigues
Publisher's note
Springer Nature remains neutral with regard to jurisdictional claims in published maps and institutional affiliations.
Rights and permissions
Springer Nature or its licensor (e.g. a society or other partner) holds exclusive rights to this article under a publishing agreement with the author(s) or other rightsholder(s); author self-archiving of the accepted manuscript version of this article is solely governed by the terms of such publishing agreement and applicable law.
Reprints and permissions
About this article
Rehman, A., Farooq, M., Lee, DJ. et al. Sustainable agricultural practices for food security and ecosystem services. Environ Sci Pollut Res 29 , 84076–84095 (2022). https://doi.org/10.1007/s11356-022-23635-z
Download citation
Received : 04 October 2021
Accepted : 10 October 2022
Published : 18 October 2022
Issue Date : December 2022
DOI : https://doi.org/10.1007/s11356-022-23635-z
Share this article
Anyone you share the following link with will be able to read this content:
Sorry, a shareable link is not currently available for this article.
Provided by the Springer Nature SharedIt content-sharing initiative
- Biological pest control
- Pollination
- Soil quality
- Carbon sequestration
- Greenhouse gas emissions
- Nutrient cycling
- Biodiversity
- Find a journal
- Publish with us
- Track your research
This page has been archived and is no longer updated
Sustainable Agriculture

History and Key Concepts

Sustainable Agriculture and the Management of Natural Resources
Sustainable agriculture and society, acknowledgements.
Feenstra, G., Ingels, C., Campbell, D. What is Sustainable Agriculture? University of California Sustainable Agriculture Research and Education Program. http://asi.ucdavis.edu/sarep/about/def
References and Recommended Reading
Altieri, M. A. Agroecology: The Science of Sustainable Agriculture. Boulder, CO: Westview Press, 1995.
Gliessman, S. R. Agroecology: Ecological Processes in Sustainable Agriculture. Boca Raton, FL: CRC Press, 2000.
Hinrichs, C. C. & Lyson, T. A. Remaking the North American Food System: Strategies for Sustainability. Lincoln, NE: University of Nebraska Press, 2008.
Flag Inappropriate


Email your Friend

- | Lead Editor: Sanjai J. Parikh

Within this Subject (20)
- Introductory (4)
- Intermediate (10)
- Advanced (6)
Other Topic Rooms
- Ecosystem Ecology
- Physiological Ecology
- Population Ecology
- Community Ecology
- Global and Regional Ecology
- Conservation and Restoration
- Animal Behavior
- Teach Ecology
- Earth's Climate: Past, Present, and Future
- Terrestrial Geosystems
- Marine Geosystems
- Scientific Underpinnings
- Paleontology and Primate Evolution
- Human Fossil Record
- The Living Primates

© 2014 Nature Education
- Press Room |
- Terms of Use |
- Privacy Notice |

Visual Browse
- Search Menu
- Computer Science
- Earth Sciences
- Information Science
- Life Sciences
- Materials Science
- Science Policy
- Advance Access
- Special Topics
- Author Guidelines
- Submission Site
- Open Access Options
- Self-Archiving Policy
- About National Science Review
- Editorial Board
- Advertising and Corporate Services
- Journals Career Network
- Dispatch Dates
- Journals on Oxford Academic
- Books on Oxford Academic

Article Contents
Properly defining sustainable agriculture, sustainable agriculture and technological development, advancing good policies for sustainable agriculture, from the household responsibility system to sustainable agriculture, from utilizing mechanization to overcoming insufficient agricultural labor, farm trade and sustainable agriculture, biotechnology and sustainable agriculture, facing climate change challenges.
- < Previous
Agriculture: science and technology safeguard sustainability
- Article contents
- Figures & tables
- Supplementary Data
Hepeng Jia, Agriculture: science and technology safeguard sustainability, National Science Review , Volume 6, Issue 3, May 2019, Pages 595–600, https://doi.org/10.1093/nsr/nwz036
- Permissions Icon Permissions
China has traditionally placed tremendous importance on agricultural research. Meanwhile, in recent years, sustainable agriculture has been increasingly highlighted in both policy agenda and the capital market. However, while terms like environmental friendliness, low carbon, organic and green agriculture have become buzzwords in the media, few meaningful discussions have been raised to examine the relationship between science and technology (S&T) development and sustainable agriculture. What's more, some environmentalists stress that sustainable agriculture should abandon modern agriculture's heavy reliance on science and industrialization, making the link between agricultural S&T and sustainable agriculture seem problematic. What is the truth? If S&T are to play an important role in advancing sustainable agriculture, what is the current status of the field? What factors have caused the sustainable development of agriculture in China? At an online forum organized by the National Science Review ( NSR ), Hepeng Jia, commissioned by NSR executive editor-in-chief Mu-ming Poo, asked four scientists in the field to examine the dynamic relationship between sustainable agriculture and agricultural S&T in the Chinese context.

Jikun Huang
Agricultural economist at Peking University, Beijing, China

Xiaofeng Luo
Agricultural economist at Huazhong Agricultural University, Wuhan, China

Jianzhong Yan
Agricultural and environmental scientist at Southwest University, Chongqing, China

Veterinary scientist at Institute of Subtropical Agriculture, Chinese Academy of Sciences, Changsha, China

Hepeng Jia (Chair)
Science communication scholar at Cornell University, Ithaca, NY, USA
Jia: In recent years, sustainable agriculture has become a hot issue in China. Meanwhile, the term is often confused with organic or green agriculture. In 2015, the State Council issued a national outline on sustainable agriculture. I guess that there should already be an authoritative definition. Let's discuss this first.
Luo: I personally think that low-carbon agriculture, organic agriculture or other concepts have emphasized different aspects of sustainable agriculture. For example, low-carbon agriculture stresses its energy-efficiency. Organic agriculture emphasizes the controlled use of chemical fertilizers and additives. Sustainable agriculture, by comparison, lies at a higher and more comprehensive level.
Yin: I think the concept of sustainable agriculture means that it realizes the balance of supply of agricultural products for contemporary human beings without destroying the resources for and the interests of future generations. It is the long-term stable development of agriculture and resources, but the key is to apply modern science and technology (S&T) to solve the bottleneck problems that restrict the sustainable development of agriculture.
No matter whether this sustainable agriculture features low-carbon agriculture or organic agriculture, the most fundamental aspect of sustainable agriculture is to adopt modern technology. —Yulong Yin
No matter whether this sustainable agriculture features low-carbon agriculture or organic agriculture, the most fundamental aspect of sustainable agriculture is to adopt modern technology. Only focusing on low-carbon agriculture or organic aspects of agriculture is not sustainable.
Jia: Prof. Yin said that technology plays a very important role in sustainable agriculture, but there are also some environmentalists who think that modern agriculture is too deeply affected by technology, which has impacted the sustainability of agriculture.
Huang: I agree with Prof. Luo and Prof. Yin. Sustainable agriculture does not only mean sustainability, but also means the growth of agriculture. Technological innovation is very important. Given the limit of water and land resources, you have to produce more products to meet food demand, you have to increase the productivity, and for certain products, the increased output must be achieved with fewer resources. Genetically modified (GM) crops are one of the many S&T innovations needed to support sustainable agriculture.
Yan: I am also interested in debates on the path of sustainable agriculture. In particular, there are some disputes between developed and developing countries on some aspects of sustainable development. Many developed countries have proposed sustainable development based on the situation of having abundant land resources. They adopt land- and capital-intensive patterns of agriculture and now they have more resources to stress environmental friendliness.
But in many developing countries, they have not come out of the so-called Malthusian trap [Editor's note: population outgrows resources and subsistence, leading to food shortages]. They still have poor people who struggle to make a living, are still destroying the environment and causing environmental pollution. Therefore, the understanding of sustainable agriculture between developed and developing countries may be different. China has some characteristics of developed countries and some characteristics of developing countries.
In the past two or three decades, China has experienced the process of the intensification of agriculture. In particular, China has been experiencing a revolution in agriculture, mainly characterized by the intensive use of labor force and capital in agriculture, for example, in vegetable farming. So, in this respect, we really have had some measures of intensive agricultural development as in developed countries.
On the other hand, the vast western part of China still has not got rid of the Malthusian trap. In these places, the problems of desertification and land loss are still very serious, and even more serious than in the past. So, in general, in terms of sustainable development, we have to adopt different paths to sustainable agriculture in different regions.
Jia: The panelists have a strong consensus that sustainable agriculture cannot be separated from modern S&T. Now let's examine how S&T innovations can promote sustainable agriculture.
Yin: I believe that achieving sustainable development of agriculture must rely on technology. At the primary level, technology can improve agricultural output, solving the contradiction between huge food demands and limited amounts of farmland.
We consume a lot of meat every day. But meat production is constrained by China's lack of land. For example, we need around 200 million tons of feed per year in China, more than the USA. Over 60% of that is imported. I believe that traditional or organic agriculture cannot fundamentally maintain China's food security and the sustainable development of agricultural restructuring.
In the past 20 years, S&T progress in animal husbandry has increased the survival rate of baby animals by 30% and improved feed conversion by 30%, and reduced nitrogen and ammonia emission by 20%, water consumption by 10% and feces production by 15%. Therefore, modern S&T play a key role in improving animal husbandry's outputs, minimizing its consumption of arable land and water resources, and reducing its pollution emissions. This certainly has contributed to the sustainable agriculture development.
However, domestic livestock and poultry farming still suffer from problems such as low feed utilization rates and epidemic disease. These lead to the inefficient use of food resources, feed waste and environmental pollution.
So, what do we do? We have to rely on modern agricultural technology. We have to improve water utilization efficiency. We must combine the farming and breeding industries. Agricultural mechanization is also very, very important. Some of the young people in our village now go to cities to work, so we have to engage in intelligent agriculture. We must make our agricultural machinery highly efficient, so that we can make our agriculture sustainable.
Luo: In the development of the entire agricultural economy, the important role of S&T goes without saying. First of all, it can compensate for the lack of resources, whether it is land or water. If you have to feed so many people, you must rely on technology under the premise of this rare land or water. Second, technology can also improve the efficiency of our agriculture. Traditionally, food security is the pursuit of the grain output. The reason why we have achieved continued grain output growth in these years is technology improvement.
For sustainable agriculture, China has some characteristics of developed countries and some characteristics of developing countries. —Jianzhong Yan
Then, I think technology is also improving our ecological environment. In recent years, China's agricultural ecological environment has been improved. This is, in large part, because technology plays a role, for example, reducing unnecessary resource consumption in agriculture.
Finally, agriculture is not the same as other industries. It is easily subject to natural disasters. There are many such unpredictable natural disasters. To reduce this risk, it is very important to rely on technology.
Huang: I would also add a little bit. Many technologies can promote the sustainable development of agriculture. Now irrigation technology, including water-saving irrigation technology, is very important. Chemical technology innovation promotes the uses of quality fertilizers and low-toxicity pesticides in China. Various bio-pesticides as well as the biological control of pests will play an important role in our sustainable development.
There are also some big improvements in agronomy, including the systematic integration of farming and animal husbandry. The application of ICTs (information and communication technologies) in agriculture is also emerging. They will have great impact on precision agriculture and more efficient use of resources. I think that the most important thing to talk about is biotechnology, because biotechnology is one of the most important technologies for promoting agricultural development, especially for sustainable agricultural development.
Jia: Sustainable agriculture needs a good system to support. Let's explore this aspect further.
Huang: Science and technology innovations are very important to sustainable agriculture, but we need a good incentive system and favorable institutional arrangements for these innovations. We have to provide better incentives for the scientists in the innovations, and appropriate incentives for farmers to use new technologies in agriculture. Generating new technology needs institutional guarantees. If you do not have a good national institutional arrangement, it is also difficult to generate and commercialize innovative technologies.

A pig farm in China as seen from outside. Facing challenges ranging from diseases like African swine fever to improving meat quality, China's animal husbandry is in urgent need of adopting modern S&T to support its sustainable development ( Courtesy of Yulong Yin ).
Jia: We all know that, in 2015, the State Council released a long-term plan for sustainable agriculture (2015–2030). Why is this important? Why do we need a State Council regulation rather than simply a ministry order? How can policies promote technology development for sustainable agriculture?
Science and technology innovations are very important to sustainable agriculture, but we need a good incentive system and favorable institutional arrangements for these innovations. —Jikun Huang
Huang: The development of sustainable agriculture has gone beyond any individual ministries. It is not simply about the environment, agricultural production or agriculture S&T. Therefore, top-level policy design and coordination are necessary.
Yin: I participated in some discussions about the development plan. It involves national food security, financial security and ecological security issues. The agricultural sector alone cannot be relied upon, nor is it completely implemented by the planning departments, nor is it solely done by the environmental agencies. The involvement of National Development and Reform Commissions (NDRC), the Ministry of Finance and the Ministry of Education are all essential. To solve sustainable developments in agriculture, we must achieve the goals set by our agricultural S&T development plan. It needs top-level design and institutional arrangements at the national level so that all agencies can participate.
Then I think the plan enacted by the State Council means that its significance is very high, especially in the background of implementing the Beautiful China task required by President Xi Jinping. When we build a beautiful China, we must do this with sustainable development of agriculture. This is the new agricultural modernization road with Chinese characteristics. Top-level policy design and implementation are a must.
Meanwhile, while China's agricultural and rural economy has made great achievements, we also face some problems, that is, the excessive development of rural resources, the high input in agricultural production, and the excessive use of natural resources, especially groundwater. Therefore, the State Council issued such a document. It is a programmatic outline and an agenda of action.
Luo: The State Council's development plan is not just one document, but it also includes the Beautiful China strategy mentioned by Prof. Yin, which involves many ministries. So, if we want to promote sustainable development of agriculture, we need to have institutional arrangements. This national agricultural sustainable development plan is a type of institutional arrangement. Second, because the situations in different regions are different, the regional sustainable development strategies are definitely not the same.
Jia: We have discussed institutional arrangements for sustainable agriculture. China's household responsibility system for farmland use [Editor's note: the system enables Chinese peasants to hold long-term farmland-use rights for decades or even longer without legally changing the literal collective land ownership] has resulted in small-scale family farms, which may result in short-sighted behavior in agriculture. Will such short-sighted behavior impact sustainable agriculture?
Huang: The household responsibility system is a basic institutional arrangement in rural China and was the greatest institutional innovation in the past. I do not agree that it resulted in short-sighted behavior. This institutional reform has provided great incentives for farmers to raise productivity and increase agricultural production. Of course, it also leads to small-scale farming systems. But there are also many other institutional innovations that have promoted land consolidation, such as the land transfer platforms and land rental markets. In fact, the usage rights of more than one-third of farmers’ contracted land are now being transferred between farmers.
China does not have the natural conditions to develop big farms as in North and South America, but the scale of land transfers is not low. Let's compare with our neighbors. Japan and South Korea have adopted private ownership of land. But their land transfers in the past century were not higher than China in the past one or two decades. So, the key is not the scale of farms but developing appropriate farm sizes, generating advanced technologies that these farms can use, and offering off-farm jobs to rural laborers so that farm sizes will expand and farmers’ incomes will rise.
Yan: I will add one more point. In the past few years, there has been a lot of comparative research in China, comparing these small family farms with large farms set up on the basis of land transfer. It was found that in Shandong Province's vegetable or tobacco planting, small family farms have higher net returns and more productivity. The reason is simple. Large farms with transferred farmland pay rent and labor costs at the price of non-agricultural workers, but small family-run farms use their own elderly and women. They don’t need to pay wages, so this small farm is completely capable of competing with the ranch.
In fact, we are currently doing a lot of investigations in Chongqing and other southwestern regions. All the farms with transferred land are losing money. After the loss, they have to find local governments to provide them with subsidies. With government subsidies, some big farm operators insisted on operating their farms but made more losses. These facts show that land transfer and the corresponding large farms are not certainly the answer to sustainable agriculture.
China does not have the land resources to encourage large-scale farms like in the USA. Then, should labor- and capital-intensive farms of appropriate sizes be the main direction of our agricultural development?
Jia: Dr Yan has raised an important aspect of China's modern agriculture, the labor- and capital-intensive middle-sized and small farms. Can we elaborate on this in the context of sustainable agriculture?
Yan: This kind of new agriculture now accounts for about one-third of the cultivated land, but its output value is very high.
Conventional agriculture accounts for two-thirds of the country's cultivated land, planting crops such as grain, cotton, and rape, but its output value only accounts for over 10%. We will further pursue this labor- and capital-intensive agriculture in the future. This is because Chinese people have changed their food consumption structure from dominantly relying on grains to consuming a high amount of meat, eggs, vegetables and fruits. This is a natural result of our higher income. This has created good opportunities for sustainable agriculture.
The increasing number of middle-class people and their higher incomes have resulted in a huge demand for high-quality organic products. We do not talk about their production amount but their output value, because the current unit price of organic products is 10 times the unit price of conventional agricultural produce.
In fact, some regions in Shandong Province have already exported this organic agricultural produce to Japan and South Korea. Their tests are very strict. It is said that some of Shandong's organic agricultural produce exports to Japan will undergo tests with more than 600 components. This type of labor- and capital-intensive small farm is transforming our agriculture. Because we now have so many middle-class people, meeting their demands will promote the transformation of agriculture into these high-value small farms.
Yin: I have a slightly different view. The household responsibility system has its own limitations, such as hindering the development of agricultural mechanization. If I only have two or three mu (1 mu = 0.16 acre) of land in my household, how can we engage in agricultural mechanization? This small farm may waste the production resources and the productivity is low.
We have been discussing land transfer. But in my hometown, much farmland is no longer planted. All the young peasants are working in cities. The land is deserted there. But in some cases, when the land can be transferred to be concentrated with one or two very capable farmers, they have incentives to cultivate the farmland, as the land concentration will not only lower costs but can also be used for finance.
Jia: Prof. Yin has raised an important point – the abandonment of farmland. In fact, it is inevitable to talk about China's urbanization here. After working in cities, young peasants will not return to the countryside. Will this have a great impact on sustainable agriculture?
Luo: Agricultural development needs a high-quality workforce. Currently, the rural labor force dramatically flows to the city, leaving the countryside with old people. I think we may need technology to solve insufficient labor quantity and quality.
At present, many regions are promoting the use of technologies, such as drones or information technology, to solve the problem of insufficient labor. The development of this smart agriculture can be a very important direction for our sustainable agriculture.
Yan: At present, the ways to solve labor outflows in different regions vary. Social services, such as providing machine sowing, machine harvesting, or socialization, are adopted to solve the problem in many parts of China. At present, the demand for labor in plains areas is greatly reduced, and seniors who remain in the countryside can meet the demand.
But in mountainous areas, the problem is very obvious. The cultivated land is far away from the residents. Therefore, in mountainous areas, farmland abandonment is particularly serious. But there are also mechanization efforts to overcome this. Small farms may hinder the application of mechanization. But in recent years in China, small and micro machines, such as handheld grass cutters and micro tractors, have been widely used. This can solve the labor insufficiency after young peasants move to cities.
In some areas where using machines is too difficult, we can simply abandon them, and they can be reforested. Abandoning marginal land is a worldwide trend. There is nothing to worry about. In fact, despite workforce loss and abandoned land, China's agricultural outputs have kept on growing in recent years.
Huang: I want to follow up the point on outflow of young labor from the countryside. This is a reality but certainly not a problem. The average age of agricultural labor in China is about 53 to 54. In the USA, the average age of farmers was 58.3 in 2012 while, in Japan, the average age is 67. This is a result of natural selection or division of labor. Senior persons generally have more comparative advantage in farming than in manufacturing or service industries, while youths have less comparative advantage in farming than other sectors. In most countries, you will find a positive association between per capita income and the age of agricultural labor.
So, the problem is not that we have older peasants left in rural areas, but how to improve their capacity to use new technologies. Don’t expect many young peasants to return to the countryside for farming.
Technological innovation can help to deal with the ageing issue in farming. For example, agricultural machines can be made easier to use for seniors.
We need technology to solve insufficient labor quantity and quality needed for sustainable agriculture. —Xiaofeng Luo

A tea farmer in the Chinese province of Hubei picking tea with a tea-picking machine. Small-scale machinery has been widely used in the Chinese countryside to solve labor shortages while improving the efficiency of sustainable agriculture ( Source: China News Service Photo ).
Another point is the difficulty in mechanization for farmland in mountain areas. While small machines can partially help to solve this problem, with higher labor costs, planting conventional crops in these areas may not have reasonable profit. I think this can be partially solved by transforming production structure from the current crops to forage grass or orchard production. In many southern mountainous areas, natural conditions are very suitable for developing grass.
Yin: Growing herbal plants may be considered as one division of these grass and timber industries. In recent years, it has developed very quickly in my hometown province of Hunan.
Yan: The central government now has subsidized agricultural mechanization, including using micro machines in mountainous farmland. Now rechargeable grass cutters are widely used in the Chinese countryside. So here we have another example of S&T innovations promoting sustainable agriculture.
Jia: Like the outflow of young agricultural labor, another major trend in agriculture in China is the massive imports of agricultural products such as soybean and corn. How will the farm trade impact on sustainable agriculture?
Huang: I want to correct one point. We are the world's largest importer of agricultural products in terms of total amounts, but China's per capita import values are one of the lowest. China imports land- and water-resource-intensive agricultural products but exports products like fruit, vegetables, fish and processed food products. The net import is only about 5% of our total food consumption, primarily soybean, sugar and dairy products. If counted in water, the amount of water needed to irrigate the imported agricultural products in 2015 was equivalent to 25% of the total irrigation water in China in that year. If counted in land, the amount of land needed to plant the imported agricultural products in 2015 was equivalent to 35% of China's total farmland. Therefore, imports substantially reduce China's pressure on scarce land and water resources, contributing to sustainable agriculture.
Yin: I basically agree with Prof. Huang. But facing the current trade war, it is necessary for China to increase its own soybean output, so that our food security can be safeguarded.
Yan: What Prof. Huang recommended is equivalent to the so-called virtual water and virtual land. With the globalization of resources and supply chains, it is reasonable for the government to study how to ‘save’ and ‘develop’ these virtual resources. For example, African and South American countries are also very eager to sell their agricultural products to us. Carefully planning these virtual resources will promote China's sustainable agriculture.
Jia: This forum was scheduled to discuss the role of agricultural biotechnology and particularly GM (genetic modification) technology in sustainable agriculture. Prof. Huang is the nation's top expert on this. Can you clarify the relationship?
Huang : GM technology has been shown to increase crop yield and lower production costs, which raises farmers’ income. With the adoption of GM technology, China can also reduce its agricultural imports. With rising agricultural production and therefore falling prices, the competitiveness of China's agriculture can be improved, which contributes to China's national food security. Because most of the current GM technologies are resistant to insects, the technologies have significantly reduced the amount of pesticides used. The biggest problem with food security now is the high residue of pesticide in food, and the development of our GM technology can promote food security by reducing pesticides.
GM technology can also improve the efficiency of all chemical use. By reducing the use of pesticides and fertilizers, GM technology will have a very important impact on our greenhouse gas emissions and mitigate the impacts of climate change.
In addition, the drought-resistant GM varieties can save water. Taken together with GM technology, we will be able to develop more resource-saving agriculture, and the increase in productivity is equivalent to saving farmland for a given output.
In fact, agricultural biotechnology is not limited to GM technology. It is estimated that the annual output value of agricultural biotechnology should have surpassed 100 billion yuan (US$14.4 billion), including animal biotech medicine, animal vaccines, biotech fertilizers, molecular breeding, ecological protection and so on. In addition to GM technologies, there are many new types of agricultural biotechnology, such as new enzymes that transform straw to feed suitable for ruminant animals through fermentation. Using corn to feed pigs, perhaps products from 1 mu of corn can only meet the food demands of one pig, but if we can transform straw to feed, we can raise five pigs using the same amount of farmland.
Jia: Given the vital importance of agricultural biotechnology, particularly GM technologies, in sustainable agriculture, why did the abovementioned 2015 national plan on sustainable agriculture not mention GM technologies?
Huang: One reason could be planning officials’ lack of knowledge on GM technologies. But more importantly, it might be influenced by public opposition. Our studies show that, in 2001, the percentage of consumers accepting GM technologies reached two-thirds, but it declined to 24% in 2012, and further down to just over 10% in 2016. More science popularization efforts for GM technologies should be made, but on the other hand, policymakers should not rely too much on public opinion to decide whether to advance GM technologies. There should be political commitment to push ahead and commercialize GM technologies given their tremendous role in raising agricultural productivity and sustainable development of agriculture.
Jia: Climate change is one of the grand challenges that human beings face. What can sustainable agriculture do for us in this aspect?
Yan: We have been studying the impact of climate change in the Qinghai–Tibet Plateau. Well, climate change may have some positive impacts on China's agriculture, and it may also have negative impacts. As the climate warms, it makes the planting of many crops in China move northward and often westward, right? In the past few years, the expansion of many crops such as glutinous rice and wheat has been very obvious.
On the other hand, the negative effects of this climate change are that there are more disasters. Many of these droughts, and droughts caused by this extreme climate in particular, have a great impact on pastoral areas! In other words, climate warming will exacerbate the prevalence of pests and diseases, making the use of pesticides increase. In addition, higher temperatures have also caused soil to lose organic matter, which has accelerated the degradation of the soil. Both the positive and negative impacts of climate change raise challenges to sustainable agriculture.
Huang: I agree with Dr Yan. Climate change has different effects in different regions in China. So simply talking about how climate change is a problem for sustainable agriculture is not enough. It has both positive and negative influences. In particular, there are management issues related to water, because no matter whether the consequence of climate change is drought or floods, they are related to water management. Therefore, we should further strengthen the utilization of water resources, improve their efficient use, and manage related issues in water resources. This is an important aspect of sustainable agriculture.
Just now we emphasized the role of technology in sustainable agriculture. Faced with the challenge brought by climate change, it is necessary to promote sustainable agriculture with technology.
Email alerts
Citing articles via.
- Recommend to Your Librarian
Affiliations
- Online ISSN 2053-714X
- Print ISSN 2095-5138
- Copyright © 2024 China Science Publishing & Media Ltd. (Science Press)
- About Oxford Academic
- Publish journals with us
- University press partners
- What we publish
- New features
- Open access
- Institutional account management
- Rights and permissions
- Get help with access
- Accessibility
- Advertising
- Media enquiries
- Oxford University Press
- Oxford Languages
- University of Oxford
Oxford University Press is a department of the University of Oxford. It furthers the University's objective of excellence in research, scholarship, and education by publishing worldwide
- Copyright © 2024 Oxford University Press
- Cookie settings
- Cookie policy
- Privacy policy
- Legal notice
This Feature Is Available To Subscribers Only
Sign In or Create an Account
This PDF is available to Subscribers Only
For full access to this pdf, sign in to an existing account, or purchase an annual subscription.
- Research Article
- Open access
- Published: 19 August 2020
Participatory research for sustainable agriculture: the case of the Italian agroecological rice network
- Elena Pagliarino ORCID: orcid.org/0000-0001-6140-3856 1 ,
- Francesca Orlando 2 ,
- Valentina Vaglia 2 ,
- Secondo Rolfo 1 &
- Stefano Bocchi 2
European Journal of Futures Research volume 8 , Article number: 7 ( 2020 ) Cite this article
16k Accesses
18 Citations
1 Altmetric
Metrics details
Since the Green Revolution, worldwide agriculture has been characterized by a typical top–down approach. The degree of autonomy, creativity, and responsibility of farmers has been limited by the continuous external inputs of chemicals, machinery, advice, subsidies and knowledge.
The issue of sustainability has brought complexity and uncertainty to this mainly linear process of innovation, steering agriculture toward alternative models. Agroecology represents an innovative paradigm of agriculture in which external inputs are minimized, and the assets of the farm are greatly valued. Agroecological production relies on the farmers’ direct management of resources and on their active engagement in the agricultural knowledge and innovation system.
This paper focuses on the experience of a group of farmers, scientists, public officials, and managers of private companies who are experimenting with agroecology in rice production in one of the most intensively farmed, profitable and environmentally sensitive areas of Italy. The partnership regularly comes together to discuss agricultural techniques and results, needs, and paths of innovation; in addition, it stimulates and takes part in research projects, following a participatory process based on co-learning and mutual responsibility. By using ethnographic methods such as direct observations and in-depth interviews, our work may contribute to understanding the role of participatory research in sustainable agriculture and what makes for good participation.
Introduction
The traditional model of innovation and its failings.
From the so-called Green Revolution, started in the 1950s, to the current period of innovations based on digital devices, worldwide agriculture has been characterized by a typical top–down transfer of technology. In this pervasive paradigm, technology is developed in the controlled environment of universities and research stations, passed on to agricultural advisors and then to farmers, who consume and apply it ([ 18 ]: 67). Technology is perceived as a commodity delivered to farmers, who have little control over its development and management [ 22 ]. The transferred technologies are uniform, standardized, and mass-produced to work almost everywhere. Standardization is applied not only to physical technologies, such as seeds, pesticides, and machinery, but also to procedures and their sequencing, with the aim of routinizing the activities of farmers, thus promoting predictable and manageable changes in rural areas ([ 18 ]: 71). Some feedback is provided by the extension agents, who turn the problems of the farmers into researchable questions, then answered by research scientists. Nevertheless, the innovation pipeline is mainly linear and one-way [ 82 ].
This system has improved the availability and quality of food per capita, ensuring food security in many areas of the world [ 72 ], and it has been a powerful tool for the diffusion of industrial agriculture [ 81 ].
While this traditional model is still practiced in many areas, its shortcomings have long been acknowledged. The reliance of farmers on suppliers of technologies, capital to buy such technologies and experts’ knowledge to be able to use them has grown, limiting their margins of autonomy and creativity in farming decisions. They have also lost control over essential resources due to the concentration of power in the mechanical, seed, chemical, processing, and distribution industries. With the introduction of advanced techniques, such as genetic engineering, nanotechnology, precision agriculture, sensors, satellites, and robotics, innovation has become increasingly sophisticated and its development even more disconnected from farmers.
Chambers, Pretty and other practitioners of the “farmer first” discourse [ 16 , 17 , 77 , 78 ] have highlighted the failure of this model in developing countries and resource-poor areas, which are more risk-prone and characterized by more complex and less controllable local conditions than the areas where the technologies and practices were actually developed.
The challenge of sustainability, posed first by the Report of the Club of Rome in 1972 and then by the Brundtland Report in 1987 and the Rio Declaration in 1992, started to be perceived as an issue only at the end of the last century [ 91 ], when it brought complexity to intensive agriculture, practiced in more developed countries. The issue of sustainability has brought to the fore the concepts of risk and uncertainty also in European agriculture. Risk and uncertainty are critical matters in agriculture and, therefore, their impact on both learning and practice needs to be taken into account. Dealing with environmental risks and developing innovations to address these risks require more inclusive ways of knowing and doing, as noted by Pimbert ([ 75 ]: 22), who stated that “more inclusive ways of knowing are required to bring together the partial and incomplete perspectives of different actors faced with uncertainty, diversity and change”. This is the reason why the participatory research approach has been incorporated into European agricultural research, increasingly oriented toward the challenge of sustainability, albeit lagging behind other sectors (for example, ecosystem management, which started soon after the Rio Declaration and Agenda 21, in 1992).
Criticism of the mechanistic process of innovation has extended to all farming systems, while a broad consensus has emerged on the links between conventional agriculture and its top–down innovation, on the one hand, and the environmental crisis, on the other hand.
The agroecological paradigm based on participation
Agroecology has been proposed as a radical alternative to the Green Revolution [ 1 , 2 , 38 , 87 , 94 ]. It represents an innovative paradigm of agriculture in which external inputs are minimized, and great value is attached to the internal resources of the farm and the territory. A systemic ecological approach is adopted in order to understand the relations between living organisms and their environment. This fosters the processes that move the agroecosystem closer to a natural, mature, relatively stable, and self-sustaining ecosystem, able to maintain productivity without external inputs [ 37 ].
Our work does not explore the issue of agroecology seen as a social movement but focuses exclusively on agroecology as a system of knowledge and innovation. In this meaning, agroecological production relies on the farmers’ direct management of resources and on their engagement in the governance of the agricultural knowledge and innovation system. Proponents of agroecology as an alternative development model argue that its potential can only be realized through participatory research and extension [ 16 , 83 , 84 , 94 , 96 ]. Cuéllar-Padilla and Calle-Collado [ 22 ] define agroecology as “the practice of science with people” and stress that participation is at the core of any single process. Agroecology implies the promotion of practices that (i) fit the local contexts in which they are implemented, (ii) foster the autonomy and skills of the communities involved (as is the case with the participatory research network discussed in our case study, whose learning and empowerment processes are presented in Section 3.2), (iii) profit from locally-produced resources, included local opinions regarding sustainability ( Ibidem ).
A young male farmer of our network explains: “It is a question of development model. So, if we choose a development model that favors indistinct, undifferentiated production—a commodity, as it is called—this leads to cost increases. The progressive increase in costs combines with stagnation in terms of value generated by the production. To deal with decreasing revenues, one must increase the surface area. This model breaks up the farming community because the land is a finite good. If ten farmers work this land today but the model forces me to expand, some farms will grow but some others will inevitably disappear. This is entrepreneurial desertification in farming. Conversely, the organic agriculture model restores the intrinsic value of what it produces because it qualifies it and, mind you, it is not a matter of profiting excessively, of setting prices that consumers can’t afford, the question is how to redistribute wealth along the production chain. Thanks to the organic system, I do this work and contribute to increasing the biodiversity of the local farming businesses.”
Agroecological research requires local-scale and action-oriented solutions to deal with technical and ecological aspects, as well as economic and sociocultural dimensions, adopting a holistic perspective on agricultural management. The research approach needs to integrate scientific and empirical knowledge throughout the process, achieving the co-production of new cross-cultural innovation [ 15 , 36 , 73 ].
A university professor of the network explains: “In traditional agronomic research, we are limited to comparing fertilizers and antiparasitic agents. We decontextualize, we only look at parcels, we compare in increasing doses, we add a witness, we add replications, we use well-documented and refined statistics, we publish, and then we entrust the best technique to the extension service. The best result obtained on the parcel must necessarily also be the best result on the farm. In case of failure, we put the blame on the farmer. This is the game. Impact is not assessed, indirect effects are not considered, especially on a territorial scale. But wasn’t agronomy born along with agriculture? Agronomy is life, creativity, the daily toil of those who work the land, it is not exclusively science. The real challenge lies in complexity. But all the actors have to be involved. It might seem like a longer path, but it is actually much shorter. It is the theory of interconnections, of evolution not based on competition but rather on cooperation.”
A male farmer says: “Farmers are researchers by nature, but with a great limitation: they don’t bother taking notes. They are not interested in writing, so they don’t bother publishing the discoveries coming from their ability to adapt during agronomy activities. In the network, instead, we had to do this. We had to take notes and then discuss them with the others, even the professors, on an equal footing.”
The European Commission has explicitly encouraged the transition to sustainable farming through interactive innovation and multi-actor approaches since 2012 [ 28 ], when the European Innovation Partnership for Agricultural Productivity and Sustainability (EIP-AGRI) and its Operational Groups were launched within the Common Agricultural Policy (CAP). Multi-actor projects and bottom-up thematic networks were also designed within the Horizon 2020 research and development (R&D) framework program. The common principle was to bring together innovation actors: farmers, advisers, researchers, businesses, NGOs, and others. The collaboration among them was supposed to make the best use of complementary types of knowledge, so as to achieve the co-creation and diffusion of solutions and opportunities that would be readily implementable in practice.
In Italy, the Ministry of Agricultural Policies [ 62 ] expressed its intention to support participatory and multi-actor projects in Action 10 of the National Strategic Plan for the Development of the Organic System, emphasising the importance of knowledge sharing, co-research and co-innovation through the involvement of various stakeholders, starting from the initial phases of research. In the call for R&D projects in organic agriculture at the end of 2018, these goals were actively pursued by requiring researchers who wished to receive financial support to include at least one farmer among their research partners and by rewarding those researchers who involved more than one farmer (Ministerial Decree no. 67374/2018).
Participatory networks have multiplied in recent years, activated as part of projects, on the basis of public co-financing. Their diffusion is strengthened by the supporting environment, that is, by the facilitation, coordination, and training processes implemented [ 34 ]. Yet, facilitating dialogue between researchers and farmers is still a priority, which will be pursued in European agricultural policy after 2020 [ 26 ].
Mansuri and Rao [ 55 ] warn that “induced” participation—that is, participation promoted through bureaucratically managed research and development interventions—requires a fundamentally different approach, one that is long-term, context sensitive, and committed to developing a culture of learning by doing. This is why it is particularly interesting to study the experience of a spontaneous, self-directed, and fairly informal, yet highly functional network that seems to be a unique case in the Italian agricultural sector.
What is true participation?
The term “participatory research” is used to refer to various approaches and methods, and it encompasses different types of participation. A systematic review of thirty-five experiences of participatory processes, with the involvement of farmers, concluded that farmers are too often considered a mere source of information to be used by researchers rather than active participants in the management and transformation of rural areas [ 57 ].
As for participatory methods, many authors stress the importance of research mechanisms and designs to bring together scientific and practical knowledge [ 22 , 35 , 50 , 56 , 65 , 99 ]. Successful participatory research, it is argued, can be achieved through a structured dialogue in which the dialectical process is encouraged by regular meetings, joint reflection, and the collective development of findings and conclusions. Nevertheless, the review by Menconi et al. [ 57 ] shows that there is no preferred scheme: every initiative has its own methods and practices and is tailor-made on the researchers’ preferences, resources, context, and project. However, simplicity of approach seems to be the best quality of any participatory activity ( Ibidem ).
As for the attitude and behavior of researchers regarding participation, the literature indicates a widespread lack of awareness, interest, time, incentives, and recognition by the current research system (e.g., [ 13 , 25 , 70 ]). Agricultural scientists have been put under growing pressure to undertake participatory research, but they do not have sufficient practice, skills, and competencies ( Ibidem ). Several authors have suggested blending various forms and intensities of stakeholders’ participation with formal agricultural research (e.g., [ 52 ]), “uniting science and participation” [ 76 ], into “compromised participation” [ 12 ], making things even more difficult in terms of designing, implementing, and monitoring participatory research.
Finally, in addition to the discussion around what participation is, some authors have questioned its very value, raising the issues of inclusion, power, and governance of participation [ 20 , 43 , 44 , 55 , 63 ].
Despite continuous attention paid to the topic, there is no consensus as to what participation means, how widespread it is, whether it is a sufficient goal in sustainable agriculture, and the extent to which it is actually inclusive.
Here, the experience of an Italian network for participatory research in agroecological rice production is presented with the aim to contribute to such ongoing debate. This paper focuses on the role of participatory research in the transition to sustainable agriculture, trying to shed light on if and why it is needed and what makes for good participation.
Study context: the difficult conversion to organic farming of the rice district in Northern Italy
Italy is the leading European producer of rice [ 31 ]. The crop is grown mainly in Northern Italy, mostly in the regions of Piedmont and Lombardy, where a rich, well-organized, and interconnected district comprises farms, processing and distribution businesses, research centers and extension services, and suppliers of chemicals, seeds, and machinery [ 14 ].
The cultivation is typically intensive monoculture, without crop rotation and with heavy chemical inputs, such as fertilizers and pesticides. The impact of rice growing on the environment tends to be considered very high, especially in terms of quality of soil and surface and ground water, with risks to human health posed by drinking contaminated water [ 45 ]. The transition to organic rice farming is perceived as a solution to ensure environmental protection, economic sustainability of the farms, consumer safety, and as a measure to mitigate climate change [ 41 , 80 ].
In Italy, organic farming is regarded as the most advanced and efficient way to develop an agroecological approach [ 68 ], and the discipline of agroecology finds concrete application in organic production, regardless of whether it is certified and remunerated on the market [ 98 ]. Hence, in the remainder of this study, the concepts of agroecology and organic farming will be used interchangeably.
The principles and approaches that should be adopted to manage organic farming systems are shown in European Commission (EC) Regulation 848/2018 (art. 6 and Annex II) [ 29 ]: limiting the use of non-renewable resources and external inputs, prohibiting the use of any product for weeding purposes, also of natural origin, and minimizing the use of organic fertilizers and pesticides, through measures to enhance soil life and its natural fertility (i.e., nourishing plants primarily through the soil ecosystem) and to prevent damage by pests and weeds, choosing appropriate resistant genotypes and crop rotation, and mechanical or physical methods. Therefore, the principles and approaches underlying organic agriculture are in line with the agroecological view of farming systems, although agroecology involves a wider approach, not limited to agronomic aspects, that overcomes any labels and certification systems. Agroecology aims not only to realize low-input farming systems, based on the exploitation of natural processes, but it also focuses on social–economical aspects, such as those related to human value, knowledge sharing, and equality in power distribution among the actors of the food supply chain. It is also true that, besides their principles, the regulations for organic agriculture allow the use of external products (EC Regulation 889/2008 [ 27 ]), which should be useful during the first period of transition to achieve a balance within the agroecosystem. However, in the real life of farms, this is often interpreted in a misleading way, and organic farming could follow the “input substitution approach” by replacing inputs permitted in conventional farming with others permitted in organic farming, which are not always very eco-friendly [ 51 , 60 , 61 ], without changing the underlying crop management approach.
Nevertheless, in our case study, organic agriculture is the basis upon which agroecological systems are generated. The organic rice farmers involved in the network are also agroecological farmers. They follow agroecological principles in relation to both (i) agronomic aspects (i.e., soil fertilization based on leguminous species and crop rotation, plant protection based on resistant genotypes, and the management of field flooding, innovative strategies for weeding without herbicides, as explained in [ 69 ]) and (ii) social aspects (i.e., group experience of knowledge sharing and mutual learning).
With the elimination of chemicals, the production of rice must be pursued through a complex process of varieties selection, crop rotation, and agronomic techniques to enhance soil and water resources and control weeds and pathogens, while also respecting the specificities of the territory. This work requires sophisticated know-how, experience, and skills that the Italian rice growers have long lost because they have been completely dependent on technology suppliers. The traditional research and advisory system is committed to ecological intensification but, due to the lack of specific funding dedicated to organic production, it has carried out few experiments on organic rice farming, mainly at the research station level [ 85 ]. The high costs of the innovative technologies developed, (e.g., mulch films and transplanting techniques, and the extreme variability of cropping systems)—depending on pedo-climatic conditions, field characteristics, and the business organization of farms—have prevented the dissemination of innovations beyond few farms. The spread of organic methods has taken place rather slowly, and organic rice production has remained niche, pursued only by a handful of pioneer farmers who, in the absence of prior knowledge, test innovative practices with a self-help and trial-and-error approach, as in Padel [ 71 ]. Organic rice cultivation was first adopted by farmers whose approach was seen as an “alternative” by the local agricultural community, i.e., biodynamic, macrobiotic, radical farmers motivated by strong environmental commitment, especially women. These farmers were initially treated with skepticism by their colleagues (as reported by [ 69 ]), sometimes even with suspicion and derision. However, their innovations were then taken up by a few pioneer farmers whose opinions are influential within the rice community, so that skepticism has now decreased, but it has not disappeared completely. This information derives from personal experiences reported by the farmers of the network. A female farmer of the network, for instance, explains that: “When the locals saw me do this work [Authors’ Note: manual work to avoid the use of herbicides], under the sun, with mosquitos all around… they thought me odd, they said: ‘that one has no brain’. That was another problem I had to deal with, being seen as a bit of an outsider. (…) It was very difficult. I struggled for many years. (…) I was heavily criticized because they saw that my business was earning much less than conventional farms—at the time, conventional farms were making good money—but I didn’t want to maximize profit, I wanted to maximize my personal expectations...”
In this context of difficult transition to organic farming, the multi-actor agroecological network analyzed here is carrying out participatory research and innovation to enhance organic methods. Exploring the values, motivations, processes, and relations of this Italian agroecological rice network is useful to understand if and how experiences of participatory research can change the trajectory of development in areas of intensive agriculture.
Our research explored the role and mechanisms of a participatory research network for the conversion to organic agriculture. We identified the following research goals:
To investigate learning processes and enabling environments;
To identify limits and opportunities of participatory research networks.
The questions that guided this study include:
Why did the farmers, researchers, and other actors join the participatory research network?
What and how do they learn within the participatory network?
Which are the limits and opportunities for the future of the network?
Methodology
This article draws on fieldwork investigating the partnership created by a group of farmers, scientists, government officials, and business managers in Northern Italy, between Lombardy and Piedmont, to research agroecological rice farming.
We integrate case study research and grounded theory, as in Andrade [ 4 ], choosing an interpretive approach [ 33 , 42 , 79 , 90 ]. We use qualitative methods, such as in-depth interviews and participant observations, constantly acknowledging the pedagogical model provided by Tracy [ 92 ] for quality issues. Twenty in-depth interviews were conducted, from January to November 2018, with the members of the network, using a biographical approach [ 66 , 89 ]. The interviews started by asking the respondents to tell their stories. They were invited to reflect on the origin and evolution of their professional experience, the moments of change and the time when they joined the network. They were also asked to say why they decided to participate in the network and to evaluate the consequences on their work and their expectations for the future. Spontaneous discussion, listening, and empathy were privileged throughout the process. The interviews were noted down, audio and video recorded with the interviewees’ permission, and later transcribed.
The functioning of the network and the relations among its members were directly observed during the partnership’s meetings, from September 2017 to December 2018. It was also possible to be involved in the informal exchange of messages among the participants via social networks and email.
Midgley [ 59 ] says that supporting evidence is often based on single case studies of intervention, and Meyer [ 54 ] points out the need to consider what is unique in each intervention. Our case study describes a small network of 28 people featuring farmers, researchers, and other actors. Other European networks have the same small number of participants, around thirty [ 40 ]. Therefore, the number of in-depth interviews (20), covering 70% of the network participants and integrated with the results of the observations made directly by the researchers during the network meetings over 16 months, appears reasonable and justifiable.
Objectives, methodologies, results, drivers of change, values, and visions were analyzed using grounded theory to develop an understanding of the processes of participation, assumption of responsibility, learning, and innovation. Grounded theory, in its latest evolution (e.g., [ 19 , 21 ]), is an interpretive method used to systematically analyze texts, such as interview transcripts and observation notes, in order to build theory concepts. This is done by reading the texts with specific questions in mind, extracting themes, and coding passages with keywords and quotes.
The narrative approach is used extensively in participatory social science, i.e., education, psychology, youth and childhood studies, geography, and land management science (for example, [ 86 ]). We found few applications in rural studies. In Phillips and Dickie [ 74 ], the narrative approach has been adopted to explore the rural future associated with climate change. Boxelaar et al. [ 10 ] explored how narrative approach can facilitate change in land management, demonstrating that this approach highlighted some of the ambiguities that existed within the project, but it did very little to change the course of the project. Kajamaa [ 47 ] shows that the narrative approach is appropriate to enrich participatory research when used in a complementary way to other ethnographic methods, such as in our case.
With the aim to explore which elements of the participatory research network support the transition to organic farming, the material was organized to fit into these categories:
Objectives, structure and functioning of the network;
Processes in the network;
Values shared;
Relations, power, and inclusion.
Results and discussion
The riso bio vero network.
The Riso Bio Vero (RBV) network brings together several organic rice farmers from the heart of the Italian rice district (provinces of Pavia, Vercelli, and Novara), as well as from outside this area. Scientists, public officials, and the managers of a company distributing organic products have also joined the network. The agricultural component of the group is not very representative of Italian farmers. According to the latest census of agriculture [ 46 ], in Italy, 30.7% of farmers are women, 2.5% are under 40, 6.2% are graduates, and only 0.8% have a degree in agriculture. In Europe [ 30 ], the first three figures are respectively: 28%, 11%, and 7.5%. In the RBV network, instead, women, young people, and graduates are well represented (respectively, 7 out of 17, 3 out of 17, and all) (Table 1 ).
The most recent data on the structure of European agriculture [ 30 ] suggest that, on average, 28% of farms across the EU are managed by women, with considerable differences among countries. In Lithuania and Latvia, nearly half of all the farms are managed by women; by contrast, in Finland, Malta, Germany, Denmark, and the Netherlands, the proportion of female farm managers does not exceed 10%. Many studies demonstrate that participatory and agroecological approaches can be gender-sensitive, i.e., able to address the issues of gender inequality and inclusion (see for example, [ 39 , 67 ]).
Only 11% of all farm holdings in the European Union (EU) are run by farmers under 40 (6.9% by farmers younger than 35 and just 4.9% by women under the age of 35) [ 30 ], and persuading more young people to begin farming is a major challenge [ 5 ]. The EU is encouraging young people to take up farming with start-up grants, income support, and benefits, such as additional training ( Ibidem ). Flament and Macias [ 32 ] highlight that a growing number of urban youths, often with a university degree, are deciding to become farmers. Described as “new peasants”, many of them choose agroecology as an alternative way to enter the food system, promoting both social and environmental sustainability. The idea of young farmers being “innovative” and turning away from traditionally intensive industrial farming models was already promoted by de Rooij in 2004 [ 23 ].
On average, only 7.5% of the current generation of European farmers have received full agricultural training, and 73.5% only have practical agricultural skills, coming from professional experience. Among farm managers, educational attainment is lower among women than men (5% versus 10% for full agricultural training and 79% versus 68% for only practical training) [ 30 ].
The RBV network was established in 2016 thanks to the coming together of a group of people who, despite knowing one another, until then had only occasionally collaborated. The intensification of their relations was linked to the opportunity, offered by the University of Milan, to organize the second international conference on Organic Rice Production (ORP 2) in Milan, on the occasion of EXPO 2015, the Universal Exposition hosted by Italy and focusing on food and agriculture. The conference was very successful; teamwork was stimulating; and the goal of continuing to work more steadily together was reinforced. The people who took part in the organization of the conference felt that they had a common vision of their work and that together they could defend and enhance their activities, even against the harsh attacks suffered by the sector. At the end of 2014, in fact, a television reportage ( Report on the national TV channel Rai3) had revealed the phenomenon of “falsi bio” (false organic producers), triggering a crisis that affected the entire rice industry, both organic and conventional, and still persists. Attacks on the image of organic rice farming played a crucial role in the decision to establish the group called “Riso Bio Vero” (True Organic Rice) to affirm the integrity of a portion of organic rice growers and their firm condemnation of fake organic producers.
A young farmer of the network explains the “false bio” phenomenon in Italy by saying:
“We are 100% organic, which is a very important choice to give the business credibility. In 2014, I was among those who fought the hardest against the issue of fake organic rice. When I started the conversion, I saw that some of my competitors basically produced in the traditional way, but then all their papers were in order to obtain the certification. This is damaging to honest organic producers, consumers, as well as to conventional producers, who choose to follow the rules and don’t give in to the golden opportunity of making easy money. Unfair competition swallows up other businesses. Both conventional and organic farmers are wiped out by those who do not comply with the rules. In 2014, together with other farmers, I decided to expose this unacceptable situation. We did it, for example, by collaborating with Report (there were many other initiatives, but Report achieved the greatest visibility). We were involved in writing the episode of the program about this issue, which became a sort of turning point in Italy’s organic rice production and, to an extent, in the organic production of other sectors too. Before that, there were thousands of hectares of organic rice cultivation that were actually farmed in the conventional way. There was no crop rotation, the embankments had no vegetation—and I have never seen land remaining bare without undergoing treatment. Since Report , the history of organic farming has changed. From then on, there has been much more attention from the institutions, from politics, born of our denunciation, of our raising awareness and rebelling, of our will to redeem the sector, especially on the part of young farmers who can’t tolerate living in such a… how can I put this… such an unfair world.”
The group’s original core included ten organic rice farmers (four from Lombardy, five from Piedmont and one from Tuscany), a professor from the University of Milan, an official from the Lombardy Region, and a representative of a company distributing organic products. Afterwards, a retired official of the Piedmont Region and a professor from the University of Pavia also joined. Both academics made available to the network their research groups, made up of technicians and young researchers.
Thanks to the participation of the University of Milan in the Riso-Biosystems national project (2017-2019), two scientists from two different public research institutions joined the network too. Furthermore, the research activity became a specific work package of the project. Although it would be very interesting to analyze the relationships between the RBV network and the rest of the partnership and the level of integration achieved, such a topic is not the subject of this study.
Subsequently, some organic rice growers became members of the network either permanently (two farmers from Piedmont) or occasionally (farmers from Veneto).
The group was founded with the aim to demonstrate that organic rice can be produced in a serious way, without circumventing the limits imposed by European regulations, which forbid the use of chemicals. The group of pioneer farmers have come together to promote their common interests, i.e., demonstrating the methods and best practices at the basis of professional organic rice production. They are all officially certified organic farmers. However, their views go beyond any labels, because they believe in the agroecological approach, which regards the farm as a living system that interacts with the environment and the socio-political structure of the territory. For these reasons, they do not consider organic farming a mere sustainable alternative to conventional farming and aim to avoid products that are permitted by organic farming regulations but not environmentally friendly. They have also focused on exposing the strategies of fake organic rice producers, which circumvent the limits imposed by the European regulations forbidding the use of chemicals. Indeed, the rice sector is particular prone to fraud since, differently from other productions, organic and conventional farms share the same irrigation system, based on a network of watercourses and channels that cross the valley of the river Po. Therefore, the auditing authorities cannot deem traces of banned chemicals in rice plants to be objective proof of forbidden treatments, since it is impossible to exclude accidental contamination through the sharing of irrigation water. Furthermore, the lack of chemical residues on the rice grain, despite repeated spraying of the plant, which is a good point for consumers, prevents the distinction between the production obtained with the organic method and that obtained with the conventional method, making organic cultivation susceptible to fraud.
Around this goal, the group began to collaborate by sharing previous knowledge and experiences. The partnership gathered latent discontent toward conventional rice cultivation and bitter frustration toward false organic farmers, channeling them into a participatory research system that would allow experimentation and innovation in agroecological rice cultivation.
Network’s role, activities, and structure
Participation in the group allows its members to share know-how and improve individual techniques, quickly adopting and adapting innovations successfully tested by others and, above all, starting a new research process “from below”. The exchange of individual experiences is very important for the site specificity of organic practices. Due to extreme variability in microclimate and soil conditions, as well as in farmers’ resources, capacities and organization, a good technique for one farm may not be feasible or suitable for another. Testing different techniques at the same time within a single context, as seen in parcel experimentation both at the farm and research station level, does not provide useful results in organic farming [ 8 , 48 , 88 ]. Vice versa, the application of the same technique to many different farms allows the growers to produce new insights and learn from one another. The first approach assumes a certain level of uniformity of cropping conditions across different farms. It transfers the results obtained from experimental trials, implying convergence of innovation through a standardized pattern of techniques, valid for different places and different times (the “funnel” scheme). Unfortunately, organic fields are unpredictably diverse, due to the reduction of external inputs that minimize possible sources of variability. Farmer-led trials reveal the constraints and benefits of different techniques by applying them to a wide range of field conditions and farm contexts and then selecting and adapting those that best respond to the specific characteristics of each farm (“folding fan” scheme). Bell and Bellon [ 6 ] explain the difference between the two approaches in terms of universalization versus generalization. The active involvement of the farmers in the research process makes it possible to experiment and adapt the same techniques to different farms, to achieve the quick and efficient generalization of best practices. Because of the extreme variability of environmental conditions among organic farms, even those where the same species are grown, the rapid dissemination of innovative results would not be feasible if the farmers were not involved—that is, if it were not supported by those who spend most of their time in the fields, carefully observing nature and its interactions with their own work, supervising the experiments and verifying their results year after year.
“Results come from individual experience, but experience comes from the exchanges among the farmers, who experiment with different techniques, each on their own land, each with their entrepreneurial approach. The mixing, discussion and reflection with the researchers and officials brings about improvements in the sector. Everyone has given and received much—this is the true strength of a network. We have become a network because we have done a lot of sharing, guided by mutual trust.” (Female farmer)
The activity of the network has allowed its members to improve existing agronomic techniques, increase and stabilize yields, and make actual discoveries, such as those regarding the allelopathic function of some species used as cover crop.
The research process is complemented by mutual assistance in the choice of machinery and suppliers, as well as in the management of the business, marketing strategies, information on regulations, and funding opportunities.
At first, discussion and collaboration among the members of the network concentrated on agronomic practices, the performance and constraints of little known agro-techniques, and issues of business administration and marketing. Then, the focus widened to include questions not strictly related to farming, e.g., measures to improve the transparency and integrity of the supply chain (critical issues and opportunities regarding both the improvement of the traditional organic certification system and alternative participative certification systems), practices to enhance plant biodiversity in the paddy fields, etc.
The governance of the network is very simple. A rice grower acts as leader of the farmer members, while a research fellow from the University of Milan serves as a bridge to the academic component and animates the entire network by taking care of overall communication. The group meets periodically, about once a month, preferably at the home of the farmers’ leader. The meetings last a whole day and include a shared lunch, for which everyone brings something that they have cooked. Regular attendance is supported by sharing meals and by common participation in other activities (e.g., training visits, trade fairs). The fact that all the participants invest a great deal of time in the network meetings and activities is not seen as a limit, but as a strength of the network.
The agenda of the meetings is set and shared by email. The researchers and farmers’ leader facilitate the discussion, which flows quite spontaneously, and use a projector to present data, results and videos, but no particular participatory method or approach is deliberately used. Sometimes, visits to one or more farms follow the discussion and help to verify the progress of the experiments undertaken directly in the field.
Outreach initiatives are carried out together with the research activity, including scientific publications authored by all the members of the network, seminars and conferences (i.e., ORP3 in Brazil in 2018 and a national conference open to all the actors of the supply chain, including the media, in Milan in 2019). The network is also preparing a manual for the identification of weeds in the paddy fields, a summary document on yields in organic rice cultivation and self-checking guidelines for the certification system.
Research process
The research process is managed through four cyclically repeating phases:
A phase of discussion concerning the issues detected in daily practice and possible experiments to investigate them.
A phase of experimentation conducted by the rice growers on their own farms but monitored by the farmers’ leader and the research fellow, who periodically visit the farmers and assist them with their technical needs, both directly in the fields and from a distance via social networks and email. At times, neighboring farmers also take part in the visits, to see the fields and give suggestions.
A collective phase of gathering, sharing, analyzing, and interpreting the results.
A phase of adoption of innovations at the farm level and identification of further critical issues.
Without knowing it, the growers are using the cycle learning process proposed by Kolb in his theory on experiential learning [ 49 ], in which concrete experience, reflective observation, abstract conceptualization, and active experimentation follow one another. Such an approach does not involve specific planning or the use of facilitating tools; rather, it centers around a reflexive, flexible, and iterative process. The action–reflection cycle helps establish a body of knowledge that is constructed and refined by the participants and represents a synthesis of the different skills brought to the partnership. A good example of this process is the research activity on plant biodiversity. During a conference, a farmer came into contact with some academics from the University of Pavia who were talking about a typical indigenous species found in the paddy fields ( Marsilea quadrifolia L.), which had been declared endangered due to massive herbicide use [ 11 ]. The farmer recognized the plant from having seen it in her fields and invited the incredulous scientists to visit her farm. The discovery triggered a research project, carried out on the land of all the farmers in the network and in the university lab, to verify the relationship between agronomic practices and plant biodiversity and enhance the ecological function of the paddy fields. It also offered the opportunity to design the brand “Marsilea rice”, to be used on the market to strengthen the identity of the group in opposition to false organic farmers. This example clearly shows how flexible the network is in its activities and scope, effectively combining a wide range of disciplines.
The members of the network are all at the same level and participate in the research and innovation process without a hierarchical approach. The academics provide their knowledge and stimulate the adoption of scientific procedures, but they are open to new forms of learning from cross-cultural exchange. They emphasize that their involvement in the network is driven by genuine interest in participatory research, curiosity about its functioning and fun and excitement in experimenting alternative forms of doing research. They admit that this research approach is not successful in terms of publications.
“Now I want to test this new approach, understand if it works, where it doesn’t work, why it works, with the clear and critical thinking of a researcher, without taking for granted that it will be a successful process. For instance, in terms of publications, it isn’t, but it is undoubtedly more interesting, fun, and exciting.” (Professor, male)
The scientists have backgrounds in agronomy, natural sciences, agricultural economy, and rural sociology, but they lack specific skills in participatory methodology. They share a commitment to participatory research that prioritizes respect, trust, and openness to experience, and their attitude is fundamental to ensure good relationships with the farmers and the other actors in the network. The researchers take the farmers’ skills very seriously to prioritize research aims and develop and validate agronomic practices. This trust is perceived by the farmers and reciprocated. Indeed, regular and direct contact between the researchers and the farmers allows them to strengthen the feeling of mutual trust that they have built.
The fact that a company distributing organic products has been present since the establishment of the network has meant that many of the farmers involved have signed a supply contract with this company. The agreement requires compliance with a set of strict cultivation guidelines deemed to be even more stringent than that required by the European organic certification regulations.
A female farmer explains: “It is an unbelievably strict contract. When you sign it, you accept being under constant monitoring, with two checks, one during the growth phase, when a rice sample is taken and analyzed, and another before the harvest—two multi-residual analyses—and then constant technical inspections. There is also a sort of protocol involving green manure or harrowing, so using cover crops or rotary tillers, but no support whatsoever.”
According to the producers, this seriousness is a guarantee for their image and is well remunerated by their buyers. So far, this economic relationship among many members of the network has not been considered an obstacle to the group’s research and innovation goals.
The network’s research activities have been funded through public and private tenders (e.g., bank foundations), and some members have supported them with their own funds. Although this is an example of bottom–up research funding, the extemporaneous and unorganized nature of such support prevents any assessment of this aspect.
Furthermore, the members have not yet taken into consideration issues of research ethics, such as confidentiality, property of innovations, and conflicts of interest.
Shared values
When the members of the network describe the values that they share, they mention a wide variety of topics, such as environmental commitment, responsible business ethics, economic rationality, aesthetics, and enhanced satisfaction in doing their job. Some common principles recur in the narratives collected through the interviews:
The members of the group are engaged in organic rice cultivation because they pursue not only economic profit, but also the protection of the environment in which they work and live, for themselves and for others.
“The radical decision of going organic, which I made a few years ago, was motivated, above all, by the situation of the market, which no longer offered any guarantee of profit or sustainability from any point of view. In my opinion, organic farming went instead in the direction of sustainability and business growth oriented toward the future. It means meeting the needs of aware consumers, producing a series of positive externalities besides the mere production of foodstuff. To me, being an organic rice producer today means being a business that yields a better type of food in many regards, provides a healthy environment, and is attentive to resources, which are not my private resources but common goods for the whole community, such as water and soil. Making this choice provides positive answers to all of these issues. This is what doing organic farming means.” (Young male farmer)
They believe that farmers must take responsibility for the environmental impact of agriculture.
They honor this commitment with courage.
They include ethics among the most important values of their activity.
“Climate change has forced us to face our responsibilities. Science is not neutral; it is not aseptic. Passion, ethics, values, ideals, and vision must be part of research. In organic farming, this is a viable path. It is not just a utopia; it is technically feasible too.” (Professor, male)
They believe that in organic farming, they can express their creativity, professionalism, and values, which were frustrated in conventional agriculture.
“Doing organic farming means doing varied and creative work. This is what organic farming requires. The seasons change every year and there is no fixed date for sowing, no fixed protocol, it changes from land to land. So, you need a lot of focus and a creative mind. Agriculture of this sort relies on everyone’s collaboration, intelligence and creativity. And everyone is important.” (Female farmer)
“The biggest difference between conventional farmers and organic farmers is that organic farmers feel peace of mind, they know that they’re doing the right thing. This is the underlying reason, they know that they are working at their best, that their cultivation methods are superior in quality, without compromises, and that there is no one to tell them what they should do, to give them chemicals. They know that they are working healthy fields, not sick fields constantly in need of chemicals for this and that.” (Female farmer)
Basing their work largely on their own abilities and resources, they feel more responsible, autonomous and free of constraints than when they used conventional methods and were highly dependent on external inputs.
“I decided to work the land with my own hands because I have always liked nature. As a child, I went to the countryside and I spent entire days observing the colors, the light, the shapes of nature. Being able to do a job that would bring me back to a place that was natural to me was the right choice. Obviously, it is not all bucolic and effortless. You are faced with all the problems of a much more difficult type of agriculture that puts you in direct correlation with nature, makes you use your brain. No technician comes along to tell you what to do. There are no technicians in organic rice farming. It’s all up to us. So, this also makes you more perceptive.” (Female farmer)
They believe that organic farming is a means of reducing costs and earning the right income for a decent life. When they practiced conventional cropping, most of their revenues were used to pay consultants and suppliers, and the margins to live with dignity were limited.
“I hope I’ll have a proud future, not a meagre one, not only in economic terms, but also from the point of view of the dignity of my work, which has something to give to everyone. I want to keep doing this with my head held high and I want those who will come after me to be able to do the same, with the same pride, the same determination, the same will to do it well.” (Young male farmer)
They find satisfaction working in contact with the land and aesthetic pleasure in the observation of nature: They believe that organic farming is the only way to preserve the beauty of nature and live in harmony with it.
“This is the land I got from my ancestors, my father and grandfather. I am proud to own it and I have always felt the responsibility of owning this land. The choice of going organic developed over many years, out of the awareness that we belong to nature and, as nature’s children, we are called upon to practice farming that respects nature, that loves it.” (Female farmer)
Their mission is to prove that organic rice cultivation can be carried out seriously and transparently.
They express their opinions and values with a very high level of emotional engagement. “Years ago, if I had had to imagine what my future business and my profession would be like, I would have never imagined, never even dreamed, that I could reach such a high level of satisfaction, creation of common work, collaboration with other farmers, with universities, with the Ministry. Not in my wildest dreams. I am so very happy.” (Female farmer)
In the network, they have created a physical, epistemic, and emotional space in which they meet and engage in shared knowledge production, free of power relations.
A young male farmer says: “During our meetings, it happens that at the start I have an opinion and, by the end, I have changed my mind completely. For someone like me, that is pretty strange. It’s not easy for me to admit that my ideas were not so good after all. This is what happens in our group. The discussions and sharing all together, each with their own opinions, allow us to come up with new, better ideas. This is possible since all points of view are equally important and no one is judged because of what they say.”
A female farmer adds: “We didn’t keep anything to ourselves, if one of us found out how to do something, they would tell the others: look, this is how you can do it. (…) I don’t necessarily say the right things. Someone else might see things differently and have the right intuition for that situation. Then, when all’s said and done, I will also agree that what that person said was right…”
Speaking about the professor who is a member of the network, another female farmer says: “He was very smart, he said: I have nothing to teach you from a technical point of view. It is you who should teach me. You know all the methods. We got on well with him, because he’s an intelligent person, he gets things right away. That’s how this participated research came about. He had twelve serious businesses to collaborate with.”
In such a space, practices and emotions are both valued and legitimated. Many of the members of the group state that they have become friends and that this has allowed them to overcome the sense of loneliness widespread among organic rice farmers, which continues to be one of the main motivations for participating in the activities of the group.
A female farmer says: “We’ve also become friends, because we have met very often, we have shared many things. We spend whole days together, so we socialize, we share our problems, the nice moments, our emotions too, like the storks on the electricity poles, the frogs hopping all around, some strange bird we saw for the first time, the selfies… (…) In my opinion, this is another step in participatory research. It counts too. It has been a big help because we don’t feel lonely… Otherwise, you know, they tell you you’re odd, you’re a fool, why should you bother when you can just spray something, since no one checks anyway… so you start feeling isolated, very much so. I think it is greatly appreciated and it is the right way forward.”
Emotions emerge as an important factor in the innovative learning process of the network, as described in Lund and Chemi [ 53 ] and Bellocchi et al. [ 7 ].
The fact that agronomic science and agricultural practice are very close has fostered their mutual understanding. They speak a common language, but what has truly brought them together is the sharing of a common mission, vision, and responsibility.
The peculiarity of the RBV network is that it is made up of people that have different degrees of authority and knowledge, and yet come together. Power differences (which inevitably exist between farmers, government officials, academics, etc.) are overcome and, although the more charismatic people act as leaders, the network is not hierarchical, since each member has put a collective goal (i.e., the research objectives) before their professional aims (i.e., profit, publications, etc.). This entails more relaxed interactions, as the spirit of collaboration seems to reduce the dynamics of power normally expressed in a competitive environment.
A young female researcher says: “I used to work in another university and I was very frustrated. The way of doing research was oriented toward competition and I didn’t like that, but I saw no alternative. That was how the system worked and I was a newcomer, I counted for nothing. Then, one day, I was at a congress, sitting next to the professor who was my thesis advisor. A colleague from our group was presenting some results, which came largely from my field work. I had worked so hard for my PhD. And this colleague was showing an article, bearing the names of all the people in the workgroup, except mine. I looked again, I thought I had to be wrong. I turned toward my professor and he said: ‘See how nasty we can be?’. I wanted to cry. But that moment made me understand that I had to change. I came here and I started working on this project, together with the farmers. I might never have a university career, but this work gives me satisfaction. I spend time in the fields with the farmers and I learn a great deal from them. We have published in international journals and we have put the names of all the farmers involved, specifically to acknowledge their contribution.”
Future of the network
The network defines itself as open and inclusive, but it has not established rules for the admission of new members, and applications to join made by other producers are assessed very carefully by the member farmers. The key requirement is to adhere to the principles of seriousness that characterize the network and, until now, this has been assessed through direct knowledge of the rice growers and their fields. During the process of inclusion of new farmers, the importance of relationships based on trust means that applicants are accepted only if they are considered “true organic”, beyond any official certification.
The network also features some public officials belonging to the institutions tasked with shaping policies for the transformation of rural areas, but so far, no initiative has been launched to stimulate a formal dialogue with these institutions.
The farmers are very directly involved in the network, appreciate the research activity and equal relationship with the researchers, and intend to formalize it in the near future. For their part, the researchers find this kind of work promising and engaging. The environmental outcomes of supporting a group of pioneering farmers involved in the difficult conversion to organic production justify the commitment of public personnel (researchers and officials), at least for now. In the future, the role of both researchers and officials will need to be redefined to avoid criticism for supporting a private group. The scaling-up of the research focus from mainly agronomic interests to the pursuit of sustainable development goals may also eventually motivate public participation. A workshop to understand if and how to incorporate the Sustainable Development Goals of Agenda 2030 [ 93 ] into the network has been conducted, but it has not led to any concrete assumption of responsibility.
Conclusions
The RBV network is a group of diverse actors from the organic rice sector participating in collective, self-planned, and self-developed research. Farmers, scientists, extension agents, government officials, and business managers are co-learning and co-producing knowledge and innovation. This public–private partnership is a voluntary, multi-year relationship that addresses the needs of the organic rice farmers, as well as those of the territory and the community, i.e., environmental issues and integrity of the supply chain.
An effective process of scientific and local knowledge sharing is taking place within the network. Cooperation is based on mutual trust and a common concern, i.e., how to shift from high-input cropping to organic farming, with the ultimate goal of protecting the environment and human well-being. The members’ active participation is mainly due to the fact that the activities carried out originate from real needs and concrete research questions.
The network follows a loosely structured agenda that allows for the continuous inclusion of new matters related to organic rice farming. In contrast to traditional research projects, which are planned in advance and leave little room for changes in goals, activities and methods, the spontaneous nature of this group generates high variability in the issues addressed, constantly reorienting its approach toward the emerging research questions.
This is a self-building group, formed around existing social relations, but inclusive and flexible: the joining of new actors (i.e., additional farmers, researchers skilled in specific topics, supply chain operators, etc.) is actively pursued through dissemination activities.
The participants show a very high degree of commitment and responsibility. The most evident sign of this is the considerable amount of time dedicated to research, both on the farms and in the regular meetings. All the members of the network are equally involved in the process of (i) defining the research questions and the activities to answer such questions, (ii) managing the research activities and the network’s organization, (iii) finding the resources needed for the research, inside and outside the network, and (iv) interpreting and evaluating the results. Such engagement is what makes them responsible, which is further confirmed by their strong motivation to disseminate the research results among other stakeholders outside the network.
Their involvement in the research process is transformative for the participants, who clearly admit that, by joining the network, they have changed their practices but also their ideas and beliefs. Such learning can create further transformations both in the sector and in the territory. Thanks to their intense communication work, the project findings are shared with other farmers and stakeholders and the network’s perspectives are brought to the attention of the institutions tasked with decisions on the transformation of rural areas. It will be interesting to follow the evolution of this network, so as to understand if it will essentially remain a group of friends engaged in collaborative research activities or if it will be able to develop into a model of innovation for the sector and an interlocutor for public decision-makers. In order to become an actor in the scientific and political debate, the network will probably need a more organized structure and include other relevant stakeholders, such as consumers, rural dwellers, and environmental NGOs.
Home and Rump [ 40 ] analyze 17 European Learning and Innovation Networks in Sustainable Agriculture (LINSAs) as part of the EU transdisciplinary research project SOLINSA. LINSAs are defined as networks of producers, consumers, experts, NGOs, SMEs, local administrations, researchers, and/or extensionists who are mutually engaged in pursuing common goals for sustainable agriculture and rural development, cooperating, sharing resources, and co-producing new knowledge by creating the right conditions for communication. Our case fits this definition perfectly. Home and Rump ( Ibidem ) recognize a wide variety of network typologies: from local scale to national or transnational; from small, simple homogenous networks to large, complex and diverse networks with multiple actors and “networks of networks”; from incremental to radical innovation; from top–down to bottom-up origin; and with several action fields, including non-food oriented, food production oriented and consumer oriented. Their study shows that LINSAs may emerge from small groups of farmers or may be inspired by individuals; they may develop as the formalization of an existing diffuse network or grow through a progressive process of co-opting local groups. Their size can vary from small (about 30 members), as in our case study, to about 100,000 farmers and 2,500 facilitators. Compared with the case studies presented by the two authors, our network has the following key characteristics:
Trans-regional scale (several regions of northern Italy);
Small dimension and simple structure;
Heterogeneous participation in terms of gender and age, but more homogeneous participation in terms of experiences and values (e.g., all the members are oriented toward the production of organic rice) and categories involved (consumers and NGOs are not present);
Commitment to both radical innovations (transition from conventional to organic rice) and incremental innovations;
Spontaneous, bottom–up origin;
Various action fields, including food production oriented, non-food oriented (environmental impact) and consumer oriented;
Low degree of formality;
Loose network with closed boundaries (participation in the network is voluntary, but the inclusion of new members appears to be contingent on sharing the same values, i.e., conventional farmers not willing to change are not accepted).
Participatory network experiences, especially for organic production, can be improved by considering the results of our analysis. In particular, in line with evidence from other studies [ 34 ], the importance of a supporting environment that facilitates and coordinates the learning processes is confirmed. What our case study highlights is that this environment can also be hardly structured or formalized. Indeed, it appears that the informal nature of the network is one of the key factors in its success.
As in Mukute and Lotz-Sisitka [ 64 ], collective learning happens when a group of people with different experiences and perspectives work together on the same issues and seek to jointly develop new knowledge or tools to address problems. As in Benton and Craib [ 9 ], in the learning process there is an emancipatory intent that is committed to changing unsatisfactory and oppressive realities, such as the socioeconomic and ethical crisis in the rice sector that started in 2014.
As Von Münchhausen and Häring [ 95 ] conclude, farmer–university networks function effectively if all their participants are considered equal partners. The findings of our research confirm the results of Home and Rump [ 40 ] who analyzed 17 networks, concluding with the identification of common factors that contribute to successful collaboration. Among these is the need to identify and build a working relationship with key partners, based on mutual trust and commitment, to strike a balance between guidance and listening, interactions and freedom, and to pursue positive and critical reflection—a fragile equilibrium that is difficult and time consuming to establish.
As in Mendez et al. [ 58 ], mutual learning takes place thanks to reciprocated trust, commitment and responsibility by all actors. These processes are favored by shared values. As a professor in our network points out, “Science is not neutral; it is not aseptic. Passion, ethics, values, ideals, and vision must be part of research.”
Mutual understanding is fostered by the use of a common language, both technical and methodological. Although applied for the first time in the network, the participatory approach has been fully espoused by its members. Despite being no experts in participation techniques, the network members understand and approve the reasons for participation.
The farmers involved in the network are well educated, unlike most farmers, and this aspect may influence their ability to speak a common language, comprehended by both the researchers and the other farmers.
The conversion to organic is often seen as a matter of procedures codified by regulations for a given period of time. For farmers, however, as the case study shows, conversion does not restrict itself to these procedures, but entails transformations that transcend any legal period and definition and have to do with the learning process that occurs in the network.
Our study results contribute to the participatory research approach by showing that personal values and attitudes are crucial. These certainly originate in the professional and human paths of the people involved, but can be developed both in education and training courses and through coaching and tutoring initiatives by other farmers and researchers who have had similar positive experiences.
Agroecology is an alternative development model to the failure of the traditional top–down innovation approach. It is said to be a knowledge intensive—as opposed to input intensive—agricultural practice [ 3 , 24 ]. Agroecology is also defined as the integration of scientific disciplines, agricultural practices, and social movements [ 97 ]. Hence, it requires an interdisciplinary approach to knowledge and pluralism in the ways of knowing. Participatory research, that is a transdisciplinary process, can therefore be seen as the right approach for the transition to agroecology. However, participatory processes need skillful researchers and farmers who have the ability to implement them and are willing to engage in the collaboration themselves. If we look at the matter from a sectoral perspective, the development of human capital receives little attention in the CAP. As highlighted by several recent studies, reforms are needed in this respect. A key suggestion that can be drawn from our case study is that of investing in the development of human capital and in the education of farmers and researchers in an integrated and coordinated way, so that they can develop skills in both agroecology practice and participatory research, designing new curricula in technical schools and universities and promoting the exchange of experiences between networks. A strong push toward education in farming is needed. Initial training is of national competence and agricultural education systems vary widely throughout the EU. But better integration between school and academic education and lifelong training is planned for the future through the European Social Fund and the CAP’s second pillar on Rural Development [ 5 ]. The future of European Participatory Research Networks can benefit from this integration. At the same time, bringing together complementary types of knowledge in a transdisciplinary approach, they can support that integration in innovative ways.
Availability of data and materials
The data supporting the findings of this study (audio and video recordings of the interviews; direct observation notes) are not publicly available, as they contain information that may compromise the privacy of those participating in the research, but are available from the corresponding author on reasonable request.
Altieri MA (1989) Agroecology: a new research and development paradigm for world agriculture. Agric Ecosystems Environ 27(1):37–46
Google Scholar
Altieri MA (2002) Agroecology: the science of natural resource management for poor farmers in marginal environments. Agric Ecosystems Environ 93(1):1–24
Altieri M, Nicholls CI (2012) Agroecology scaling up for food sovereignty and resiliency. In: Lichtfouse E (ed) Sustainable Agriculture Reviews, vol 11, pp 1–29
Andrade AD (2009) Interpretive research aiming at theory building: adopting and adapting the case study design. Qual Rep 14(1):42–60
Augère-Granier, M.L. 2017. Agricultural education and lifelong training in the EU. European Parliamentary Research Service [WWW] https://www.europarl.europa.eu/RegData/etudes/BRIE/2017/608788/EPRS_BRI (2017)608788_EN.pdf (visited on 02/06/2020).
Bell MM, Bellon S (2018) Generalization without universalization: towards an agroecology theory. Agroecol Sustainable Food Syst 42(6):605–611
Bellocchi A, Quigley C, Otrel-Cass K (2017) Exploring emotions, aesthetics and wellbeing in science education research. Springer Cultural Studies of Science Education 13
Bengtsson J, Ahnström J, Weibull AC (2005) The effects of organic agriculture on biodiversity and abundance: a meta-analysis. Journal of applied ecology 42(2):261–269
Benton T, Craib I (2001) Critical realism and the social sciences. In Benton, T. and Craib, I. (eds.) Philosophy of science. The Philosophical Foundations of Social Thought, Palgrave Macmillan, Basingstoke, pp 119–139
Boxelaar L, Paine M, Beilin R (2007) Change management and complexity: the case for narrative action research. The Journal of Agricultural Education and Extension 13(3):163–176
Bruni I, Gentili R, De Mattia F, Cortis P, Rossi G, Labra M (2013) A multi-level analysis to evaluate the extinction risk of and conservation strategy for the aquatic fern Marsilea quadrifolia L. in Europe. Aquatic botany 111:35–42
Buhler W, Morse S, Arthur E, Bolton S, Mann J (2002) Science, agriculture, and research: a compromised participation? Earthscan, London
Caister K, Green M, Worth S (2011) Learning how to be participatory: an emergent research agenda. Action Res 10(1):22–39
Caraveli H (2000) A comparative analysis on intensification and extensification in Mediterranean agriculture: dilemmas for LFAs policy. J Rural Stud 16(2):231–242
Carolan MS (2006) Sustainable agriculture, science and the co-production of ‘expert’ knowledge: the value of interactional expertise. Local Environment 11(4):421–431
Chambers R, Pacey A, Thrupp LA (eds) (1989) Farmer first: farmer innovation and agricultural research. Intermediate Technology Publications, London
Chambers R (1994) The origins and practice of participatory rural appraisal. World Development 22(7):953–969
Chambers R (1997) Whose reality counts? Putting the first last. ITDG Publishing, London
Charmaz K (2006) Constructing grounded theory: a practical guide through qualitative analysis. Sage, London
Cooke B, Kothari U (eds) (2001) Participation: the new tyranny? Zed Books, London
Corbin J, Strauss A (2015) Basics of qualitative research: techniques and procedures for developing grounded theory. Sage Publications, Thousand Oaks
Cuéllar-Padilla M, Calle-Collado A (2011) Can we find solutions with people? Participatory action research with small organic producers in Andalusia. Journal of Rural Studies 27:372–383
De Rooij, S. 2004. Young farmers in Europe: opting for innovation. LEISA INDIA Magazine on Low External Input Sustainable Agriculture 6(2):24-26.
De Schutter O (2010) Report submitted by the Special Rapporteur on the right to food, United Nations General Assembly, Human Rights Council, Sixteenth session, United Nations: New York
Edwards-Jones G (2001) Should we engage in farmer-participatory research in the UK? Outlook on Agric 30(2):129–136
EIP-AGRI, 2020. Research needs from practice 2020. EIP-AGRI [WWW] https://ec.europa.eu/eip/agriculture/sites/agri-eip/files/eip-agri_report_research_needs_from_practice_2020_en.pdf (visited on 02/06/2020).
European Commission. 2008. Commission Regulation (EC) No 889/2008 of 5 September 2008 laying down detailed rules for the implementation of Council Regulation (EC) No 834/2007 on organic production and labelling of organic products with regard to organic production, labelling and control [WWW] https://eur-lex.europa.eu/legal-content/EN/TXT/PDF/?uri=CELEX:32008R0889&from=EN (visited on 03/06/2020).
European Commission. 2012. Communication from the Commission to the European Parliament and the Council on the European Innovation Partnership ‘Agricultural Productivity and Sustainability’ Brussels: European Commission [WWW] ec.europa.eu/eip/agriculture/sites/agri-eip/files/communication_on_eip_-_en.pdf (visited on 12/06/2018).
European Union. 2018. Regulation (EU) 2018/848 of the European Parliament and of the Council of 30 May 2018 on organic production and labelling of organic products and repealing Council Regulation (EC) No 834/2007 [WWW] https://eur-lex.europa.eu/legal-content/EN/TXT/PDF/?uri=CELEX:32018R0848&from=EN (visited on 02/06/2020).
Eurostat. 2016. Agriculture, forestry and fishery statistics [WWW] https://ec.europa.eu/eurostat/documents/3217494/7777899/KS-FK-16-001-EN-N.pdf/cae3c56f-53e2-404a-9e9e-fb5f57ab49e3 (visited on 03/06/2020).
FAO (2016) FAOSTAT Food and agriculture data. FAO, Rome [WWW] www.fao.org/faostat (visited on 29/04/2018)
Flament SO, Macias B (2015) New peasants moving back to rural areas. Farming Matter 31(2):12–21
Flyvbjerg B (2006) Five misunderstandings about case-study research. Qual Inq 12(2):219–245
Fotheringham, J., Hetherington, A., Kobilsky, A., Rohmer, B., Chever, T., Renault, C., Romieu, V., Carillo, J., Giambenedetti, G., Vukovic, M., Collison, M., and Kuehnemund, M. 2016. Evaluation study of the implementation of the European Innovation Partnership for Agricultural Productivity and Sustainability. Final Report. European Commission: Brussels [WWW] https://op.europa.eu/en/publication-detail/-/publication/3f035a53-e9dc-11e6-ad7c-01aa75ed71a1 (visited on 02/06/2020).
Funtowicz S, Ravetz J (1993) Science for the post-normal age. Futures 25(7):739–755
Gabathuler E, Bachmann F, Kläy A (2011) Reshaping rural extension. In: Learning for sustainability (LforS) – an integrative and learning-based advisory approach for rural extension with small-scale farmers. Margraf Publishers GmbH, Weikersheim
Gliessman SR (1995) Sustainable agriculture: an agroecological perspective. Adv Plant Pathol 11:45–57
Gliessman SR (2008) Agroecology: ecological processes in sustainable agriculture. Ann Arbor Press, Chelsea
Gomez LF, Ríos-Osorio LA, Eschenhagen-Durán ML (2016) Key concepts of agroecology science. A systematic review. Trop Subtrop Agroecosystems 19:109–117
Home R, Rump N (2015) Evaluation of a multi-case participatory action research project: the case of SOLINSA. The Journal of Agricultural Education and Extension 21(1):73–89
Gomiero T, Pimental D, Paoletti MG (2011) Environmental impact of different agricultural management practices: conventional vs. organic agriculture. Crit Rev Plant Sci 30(1-2):95–124
Guba EG, Lincoln YS (1994) Competing paradigms in qualitative research. In: Denzin NK, Lincoln YS (eds) Handbook of qualitative research. Sage Publications, Thousand Oaks, pp 105–117
Guijt I, Shah MK (eds) (1998) The myth of community: gender issues in participatory development. Practical Action Publishing, England
Hickey S, Mohan G (2004) Participation - from tyranny to transformation. Zed Books, London
ISPRA (2018) Rapporto nazionale pesticidi nelle acque − dati 2015-2016. Edizione 2018. ISPRA, Roma
ISTAT. 2010. 6° Censimento generale dell’agricoltura. ISTAT, Roma [WWW] http://censimentoagricoltura.istat.it (visited on 29/04/2018).
Kajamaa A (2012) Enriching action research with the narrative approach and activity theory: analyzing the consequences of an intervention in a public sector hospital in Finland. Educational Action Research 20(1):75–93
Kravchenko AN, Snapp SS, Robertson GP (2017) Field-scale experiments reveal persistent yield gaps in low-input and organic cropping systems. Proceedings of the National Academy of Sciences 114(5):926–931
Kolb DA (1984) Experiential learning: experience as the source of learning and development. Prentice Hall, Englewood Cliffs
Lawrence DN, Christodoulou N, Whish J (2007) Designing better on-farm research in Australia using a participatory workshop process. Field Crops Res 104:157–164
Levidow L, Pimbert M, Vanloqueren G (2014) Agroecological research: conforming — or transforming the dominant agro-food regime? Agroecol Sustainable Food Syst 38(10):1127–1155
Lilja N, Bellon M (2008) Some common questions about participatory research: a review of the literature. Development in Practice 18(4–5):479–488
Lund B, Chemi T (eds) (2015) Dealing with emotions: a pedagogical challenge to innovative learning. Sense Publishers, Rotterdam
Meyer J (2000) Evaluating action research. Age Ageing 29(2):8–10
Mansuri G, Rao V (2013) Localizing development: Does Participation Work? The World Bank, Washington
Martin A, Sherington J (1997) Participatory research methods – implementation, effectiveness and institutional context. Agricultural Systems 55(2):195–216
Menconi ME, Grohmann D, Mancinelli C (2017) European farmers and participatory rural appraisal: a systematic literature review on experiences to optimize rural development. Land Use Policy 60:1–11
Mendez VE, Caswell M, Gliessman SR, Cohen R (2017) Integrating agroecology and participatory action research (PAR): lessons from Central America. Sustainability 9:705
Midgley G (2011) Theoretical pluralism in systemic action research. Systemic Practice and Action Research 24(1):1–15
Mier M, Cacho TG, Giraldo OF, Aldasoro M, Morales H, Ferguson BG, Rosset P, Khadse A, Campos C (2018) Bringing agroecology to scale: key drivers and emblematic cases. Agroecol Sustainable Food Syst 42(6):637–665
Migliorini P, Gkisakis V, Gonzalves V, Raigón MD, Bàrberi P (2018) Agroecology in Mediterranean Europe: genesis, state and perspectives. Sustainability 10(8):2724
Mipaaf (Ministero delle politiche agricole alimentari e forestali). 2016. Piano strategico nazionale per lo sviluppo del sistema biologico [WWW] https://www.politicheagricole.it/flex/cm/pages/ServeBLOB.php/L/IT/IDPagina/10014 (visited on 12/06/2018).
Mosse D (2004) Cultivating development: an ethnography of aid policy and practice. Pluto Press, London
Mukute M, Lotz-Sisitka H (2012) Working with cultural-historical activity theory and critical realism to investigate and expand farmer learning in Southern Africa. Mind, Culture, and Activity 19:342–367
Nabasa J, Rutwara G, Walker F, Were C (1995) Participatory rural appraisal: principles and practicalities. Natural Resources Institute, Chatam
Olagnero M (2005) Vite nel tempo. La ricerca biografica in sociologia. Carocci, Roma
Oliver B (2016) “The earth gives us so much”: agroecology and rural women's leadership in Uruguay. Cult Agric Food Environ 38(1):38–47
Organic Action Network Italia. 2017. Carta del biologico di Bergamo. Il modello biologico per una produzione agricola e un consumo sostenibili. Organic Action Network Italia [WWW] http://www.anabio.it/uploads/article/cartadelbiologicodibergamo-92d8dfefbd.pdf (visited on 29/04/2018).
Orlando F, Alali S, Vaglia V, Pagliarino E, Bacenetti J, Bocchi S (2020) Participatory approach for developing knowledge on organic rice farming: management strategies and productive performance. Agric Syst 178:102739
Ortolani L, Bocci R, Bàrberi P, Howlett S, Chable V (2017) Changes in knowledge management strategies can support emerging innovative actors in organic agriculture: the case of participatory plant breeding in Europe. Org Farming 3(1):20–33
Padel S (2001) Conversion to organic farming: a typical example of the diffusion of an innovation? Sociologia Ruralis 41(1):40–61
Patel R (2012) The long green revolution. J Peasant Stud 40(1):1–63
Pence RA, Grieshop JI (2001) Mapping the road for voluntary change: partnerships in agricultural extension. Agric Hum Values 18(2):209–217
Phillips M, Dickie J (2014) Narratives of transition/non-transition towards low carbon futures within English rural communities. J Rural Stud 34:79–95
Pimbert M (2009) Towards food sovereignty: reclaiming autonomous food systems. International Institute of Environment and Development, London
Pound B, Snapp S, McDougall C, Braun A (eds) (2003) Managing natural resources for sustainable livelihoods: uniting science and participation. Earthscan Publications, London
Pretty NJ (1995) Participatory learning for sustainable agriculture. World Development 23(8):1247–1263
Pretty J (2002) Agri-culture: reconnecting people, land and nature. Earthscan Publications, London
Ragin CC, Becker HS (eds) (1992) What is a case? Exploring the foundations of social inquiry. Cambridge University Press, Cambridge
Reganold JP, Wachter JM (2016) Organic agriculture in the twenty-first century. Nature Plants 2(2):15221
Röling N (1988) Extension science: information systems in agricultural development. Cambridge University Press, New York
Röling N, Engel P (1990) The development of the concept of agricultural knowledge information systems (AKIS): implications for extension. In: Rivera WM, Gustafson DJ (eds) Agricultural extension: Worldwide institutional evolution and forces for challenge. Elsevier, Amsterdam
Röling N, Jiggins J (1998) The ecological knowledge system. In: Röling N, Wagemakers MAE (eds) Facilitating sustainable agriculture: Participatory learning and adaptive management in times of environmental uncertainty. Cambridge University Press, Cambridge
Röling N, Wagemakers MAE (eds) (1998) Facilitating sustainable agriculture: participatory learning and adaptive management in times of environmental uncertainty. Cambridge University Press, Cambridge
Romani M, Beltarre G, Tabacchi M (2007) Organic rice farming. Regione Lombardia, Milan
Savin-Baden M, Van Niekerk L (2007) Narrative inquiry: theory and practice. J Geogr High Educ 31(3):459–472
Sevilla-Guzmán E, Woodgate G (1997) Sustainable rural development: from industrial agriculture to agroecology. In: Redclift M, Woodgate G (eds) The international handbook of environmental sociology. Edward Elgar, Cheltenham
Shennan C, Krupnik TJ, Baird G, Cohen H, Forbush K, Lovell RJ, Olimpi EM (2017) Organic and conventional agriculture: a useful framing? Annual Review of Environment and Resources 42:317–346
Siciliano E (1998) Approccio biografico. In: Melucci A (ed) Verso una sociologia riflessiva. Il Mulino, Bologna
Stake RE (1995) The art of case study research. Sage Publications, Thousand Oaks
Tilman D, Cassman KG, Matson PA, Naylor R, Polasky S (2002) Agricultural sustainability and intensive production practices. Nature 418:671–677
Tracy SJ (2010) Qualitative quality: eight “big-tent” criteria for excellent qualitative research. Qualitative Inquiry 16(10):837–851
United Nations. 2015. Transforming our world: the 2030 agenda for sustainable development [WWW] https://sustainabledevelopment.un.org/content/documents/21252030%20Agenda%20for%20Sustainable%20Development%20web.pdf (visited on 5/4/2018).
Uphoff N (ed) (2002) Agroecological innovations: increasing food production with participatory development. Earthscan Publications, London
Von Münchhausen S, Häring AM (2012) Lifelong learning for farmers: enhancing competitiveness, knowledge transfer and innovation in the eastern German state of Brandenburg. Stud Agric Econ 114:86–92
Warner KD (2008) Agroecology as participatory science emerging alternatives to technology transfer extension practice. Sci Technol Hum Val 33(6):754–777
Wezel A, Bellon S, Dore T, Francis C, Vallod D, David C (2009) Agroecology as a science, a movement and a practice. A review. Agronomy Sustainable Dev 29:503–515
Wezel A, Goette J, Lagneaux E, Passuello G, Reisman E, Rodier C, Turpin G (2018) Agroecology in Europe: research, education, collective action networks, and alternative food systems. Sustainability 10:1214
Zuber-Skerrit O (2001) Action learning and action research: paradigm, praxis and programs. In: Sankara S, Dick B, Passfield R (eds) Effective Change Management through Action Research and Action Learning: Concepts, Perspectives. Processes and Applications. Southern Cross University Press, Lismore, pp 1–20
Download references
Acknowledgements
The authors acknowledge with gratitude the active involvement of the Riso Bio Vero network members and their willingness to tell their stories and share their thoughts. The interpretations in this article remain the authors’ own.
This study was carried out as part of the Riso-Biosystems three-year project (2017-2019), funded by the Italian Ministry of Agriculture, Food and Forestry Policies to study and promote organic rice. The funding body does not have any role in the design of the study, in the collection, analysis, and interpretation of the data and in the writing of the manuscript.
Author information
Authors and affiliations.
Research Institute on Sustainable Economic Growth (Cnr-Ircres), Italian National Research Council, Moncalieri (Turin), Italy
Elena Pagliarino & Secondo Rolfo
Department of Environmental Science and Policy, University of Milan, Milan, Italy
Francesca Orlando, Valentina Vaglia & Stefano Bocchi
You can also search for this author in PubMed Google Scholar
Contributions
All authors contributed to the conception and design of the work; EP and FO devoted themselves to the acquisition, analysis, and interpretation of data; EP have drafted the work and all authors substantively revised it. The authors read and approved the final manuscript.
Corresponding author
Correspondence to Elena Pagliarino .
Ethics declarations
Ethics approval and consent to participate.
This study was conducted in compliance with the CNR Code of Conduct of 10/20/2017, whose observance is supervised by the Institute Director, and in compliance with the University of Milan’s Code of Ethics and for Research Integrity, issued by Rectoral Decree 224/2019 of 18/01/2019.
Consent for publication
Not applicable.
Competing interests
The authors declare that they have no competing interests.
Additional information
Publisher’s note.
Springer Nature remains neutral with regard to jurisdictional claims in published maps and institutional affiliations.
Rights and permissions
Open Access This article is licensed under a Creative Commons Attribution 4.0 International License, which permits use, sharing, adaptation, distribution and reproduction in any medium or format, as long as you give appropriate credit to the original author(s) and the source, provide a link to the Creative Commons licence, and indicate if changes were made. The images or other third party material in this article are included in the article's Creative Commons licence, unless indicated otherwise in a credit line to the material. If material is not included in the article's Creative Commons licence and your intended use is not permitted by statutory regulation or exceeds the permitted use, you will need to obtain permission directly from the copyright holder. To view a copy of this licence, visit http://creativecommons.org/licenses/by/4.0/ .
Reprints and permissions
About this article
Cite this article.
Pagliarino, E., Orlando, F., Vaglia, V. et al. Participatory research for sustainable agriculture: the case of the Italian agroecological rice network. Eur J Futures Res 8 , 7 (2020). https://doi.org/10.1186/s40309-020-00166-9
Download citation
Received : 09 October 2019
Accepted : 03 August 2020
Published : 19 August 2020
DOI : https://doi.org/10.1186/s40309-020-00166-9
Share this article
Anyone you share the following link with will be able to read this content:
Sorry, a shareable link is not currently available for this article.
Provided by the Springer Nature SharedIt content-sharing initiative
- Rice farming
- Agroecology
- Participatory research

An official website of the United States government
The .gov means it’s official. Federal government websites often end in .gov or .mil. Before sharing sensitive information, make sure you’re on a federal government site.
The site is secure. The https:// ensures that you are connecting to the official website and that any information you provide is encrypted and transmitted securely.
- Publications
- Account settings
Preview improvements coming to the PMC website in October 2024. Learn More or Try it out now .
- Advanced Search
- Journal List
- Metabolites

The Importance of Microorganisms for Sustainable Agriculture—A Review
In the face of climate change, progressive degradation of the environment, including agricultural land negatively affecting plant growth and development, endangers plant productivity. Seeking efficient and sustainable agricultural techniques to replace agricultural chemicals is one of the most important challenges nowadays. The use of plant growth-promoting microorganisms is among the most promising approaches; however, molecular mechanisms underneath plant–microbe interactions are still poorly understood. In this review, we summarized the knowledge on plant–microbe interactions, highlighting the role of microbial and plant proteins and metabolites in the formation of symbiotic relationships. This review covers rhizosphere and phyllosphere microbiomes, the role of root exudates in plant–microorganism interactions, the functioning of the plant’s immune system during the plant–microorganism interactions. We also emphasized the possible role of the stringent response and the evolutionarily conserved mechanism during the established interaction between plants and microorganisms. As a case study, we discussed fungi belonging to the genus Trichoderma . Our review aims to summarize the existing knowledge about plant–microorganism interactions and to highlight molecular pathways that need further investigation.
1. Introduction
Traditional agricultural techniques, such as chemical fertilizers, pesticides, fungicides, and herbicides, enable the protection of crop plants against pathogens and ensure better yield. Chemical compounds present in agricultural chemicals are harmful to the environment and cause soil, atmosphere, and water pollution [ 1 ]. These compounds are the reason for the extinction of fish [ 2 ], bees [ 3 ], and plants [ 4 , 5 ], and pose a threat to the biodiversity of soil bacterial [ 6 , 7 , 8 ] and fungal communities [ 9 ]. Chemical plant protection products negatively affect agricultural soils, i.e., they change soil physical properties, (e.g., texture, permeability, porosity), they disturb the cycle of the elements, such as phosphorus and nitrogen, and they decrease the complexity of soil microbiome [ 10 ]. In the face of a growing world population and increased demand for food both in terms of food quantity and food quality, the usage of bioinoculants, i.e., biofertilizers to increase the yield and biopesticides to protect plants, is the future of agriculture. Bioinoculants comprised of living or dormant microbes that are able to promote plant growth and development are called PGPM (plant growth-promoting microorganisms) and have great potential not only for enhancing plant yield but also for remediation of degraded soils [ 11 , 12 , 13 ]. Bioinoculants are cost-effective and environmental-friendly approaches in agriculture [ 14 ]. The first step in a bioinoculant formulation is the isolation and identification of a microbe. The further potential of a particular microorganism for plant growth promotion needs to be verified, and this ability should be confirmed in laboratory and field conditions. Moreover, potential risks to other organisms, such as animals and natural soil microbiomes, should be also determined [ 15 ]. The best-known examples of PGPM are mycorrhizal fungi and bacteria belonging to Rhizobium; however, plant growth-promoting microorganisms are found among varied taxa of bacteria, fungi, and algae [ 16 ]. In Table 1 , several examples of PGPM are shown.
Examples of plant growth-promoting microorganisms. The effect of PGPM on plants and, if known, the mode of action of microorganisms is also included.
One of the major drawbacks of the application of bioinoculants is the fact that the number of bioinoculants tested in laboratory/greenhouse conditions fail in the field trials. This is mostly because microbes introduced to the environment have to compete for a niche with native microorganisms in order to attain sufficient abundance. The ability of introduced microbes to survive and thrive is variable and significantly depends on environmental conditions including temperature, rainfall, and soil type, as well as on interactions with the host plant and other organisms. A good example of this dependence is the field trial that showed that the promotion of plant growth by subtropical strains of Azotobacter chroococcum and Azospirillum brasilense was observed only when used in the same type of climate and not in the alpine region with a temperate climate [ 80 ]. Moreover, it was demonstrated that the high biodiversity of the native soil microbiome has a negative impact on the survivability of applied bioinoculants, i.e., there is a negative correlation between the diversity of the soil microbes and the survival rate of the introduced strain [ 81 ]. Therefore, when the native microbiome biodiversity is low, the chance for a new strain to thrive is higher which corresponds with better availability of nutrients and reduced competition among microorganisms for niche [ 82 , 83 , 84 ]. Moreover, several studies showed that PGPM that promotes the growth of a particular plant species might not be beneficial for other species of plants. For example, fungi belonging to Penicillium sp. and Trichoderma sp. showed diversified effectiveness in enhancing the growth of varied wheat ( Triticum aestivum L.) cultivars [ 85 ]. Similarly, the effect of inoculation of winter and spring varieties of oilseed rape ( Brassica napus L.) with different strains of PGPM on the germination rate and growth of seedlings depended on the plant variety [ 86 ]. On the other hand, different strains of the same microbial species might differ significantly in their ability to promote plant growth and development. For example, Znajewska et al. [ 59 ] showed that seven Trichoderma viride isolates had various effects on winter rapeseed germination and growth promotion depending on the used strain.
Microorganisms colonize not only roots but also other plant tissues and organs including stems, leaves, flowers, seeds, and fruits. The aerial part of plants colonized by microbes is called the phyllosphere, whereas the rhizosphere is the soil adjacent to the root [ 87 ]. In contrast to the rhizosphere, the above-ground parts of plants are scarce in water and nutrients. Only a small number of microorganisms that reach the surface of the plant will land on beneficial spots and will have conditions to survive [ 88 ]. As a consequence, the number of microorganisms living in the rhizosphere is much higher than in the phyllosphere. Microbes are present at every stage of plant development, from seed to fully developed plant producing a new generation of seeds [ 89 ]. Some microorganisms live on the surfaces of plant organs, i.e., epiphytes whereas others are able to colonize the internal tissues of plants, i.e., endophytes [ 90 , 91 ].
Plant growth-promoting microorganisms stimulate plant growth and development through various direct and indirect mechanisms ( Figure 1 , Table 1 ). Production of phytohormones [ 92 , 93 ], nitrogen assimilation [ 94 ], solubilization, and mineralization of macro- and micro-elements [ 95 , 96 ], and modulation of the endogenous level of ethylene in plants tissues [ 97 ] are examples of direct mechanisms. Examples of indirect mechanisms are inhibition of pathogens growth through antibiosis [ 98 ], secretion of lytic enzymes [ 99 ], and competition, e.g., via siderophores production [ 100 ], induction/inhibition of plant genes expression [ 101 ], induction of plant immune response [ 102 ], and manipulation of plant microbiome composition [ 103 ]. This work aims to summarize the current knowledge about the interactions of plants with beneficial microbes, and how those interactions affect the overall health of the plant. For further development of environmental-friendly methods of plant cultivation, is it crucial to deeply understand the molecular mechanisms underneath (i) the recruitment of useful microbes by plants, (ii) the interactions among microorganisms, and (iii) the plant-microorganism interplay. The interactions between plants and microorganisms can be divided into three types, i.e., interactions are either neutral, negative, or positive in their effects on the host plant. This review focuses exclusively on positive interactions and mechanisms underneath those interactions. In this work, we also discuss the role of stringent responses in interactions between plants and microorganisms.

Multidimensional network of interactions between plants and PGPM, between plants and pathogens, and between PGPM and pathogens.
2. Rhizosphere and Root Exudates
The rhizosphere is defined as “the field of action or influence of a root”, i.e., it is soil adjacent to the roots which are influenced by root exudates that are the mixture of several compounds produced and secreted by roots [ 104 ]. The main components of root exudates are water, enzymes, amino acids, nucleotides, vitamins, organic acids, fatty acids, sugars, phenolic compounds, anions, volatile organic compounds (VOCs), polysaccharides, and proteins [ 105 , 106 , 107 , 108 , 109 , 110 , 111 , 112 ]. The composition of exudates varies depending on the plant species, and even phenotype; it changes during plant development [ 107 , 113 ] and it is dependent on environmental conditions, such as temperature [ 114 ], light [ 115 ], the amount of nutrients [ 116 , 117 , 118 , 119 ], and stress factors [ 120 , 121 , 122 ]. Moreover, the type and composition of soil can also affect the composition of root exudates [ 123 ]. For instance, plants that grow in soil deprived of nitrogen probably do not secrete extra amino acids or proteins to the rhizosphere [ 119 ]. Root exudates are also an important source of elements present in the soil. It was estimated that about 10–44% of carbon compounds [ 124 ] and about 10–16% of nitrogen compounds [ 125 ] synthetized by the plant is secreted to the rhizosphere. In legumes, the rhizodeposition of nitrogen is estimated between 4% and 70%, depending on the plant species [ 126 ].
The rhizosphere is the hotspot of plant–microorganism interactions. Interactions between those organisms have a direct influence on the availability of soil nutrients for plants [ 95 , 127 , 128 , 129 , 130 ] and on plant tolerance toward biotic and abiotic stresses [ 131 , 132 , 133 , 134 , 135 ]. Exudates are a rich source of carbon and other nutrients and, therefore, the abundance of microorganisms in the rhizosphere can be up to a hundred times greater than in the bulk soil [ 136 ]. Moreover, root exudates allow plants to communicate with rhizosphere microorganisms and affect their behavior through the secretion of various signaling molecules [ 137 , 138 , 139 , 140 ]. For instance, barley ( Hordeum vulgare L.) in response to infection by the fungus Pythium ultimum, secretes increased amounts of organic and phenolic acids, which activates the expression of the phlA gene in the endophytic bacterium Pseudomonas fluorescens CHA0. Hydroxymethylglutaryl-CoA synthase encoded by the phlA gene is involved in the synthesis of DAPG (1,4-diacetylphloroglucinol) that has antifungal activity [ 98 ]. Zhang et al. [ 141 ] demonstrated the role of organic acids in root exudates of cucumber ( Cucumis sativus L.) and banana ( Musa acuminata Colla). The citric acid present in cucumber root exudates attracted Bacillus amyloliquefaciens (isolated from cucumber rhizosphere) and B. subtilis (isolated from banana rhizosphere). Moreover, it induced the formation of the B. amyloliquefaciens biofilm. Fumaric acid present in banana root exudates promoted biofilm formation of both tested strains. Biofilm is a coherent multicellular structure embedded in a self-produced extracellular matrix that can be formed on the surface of plant organs. Members of biofilm are better protected from environmental factors; their nutritional status is enhanced and, therefore, the survival rate of members of biofilm is much higher than that of single bacteria cells, as reviewed in [ 142 ]. Another example of a molecule allowing plant–PGPM communication is polyamines present in root exudates, which inform rhizospheric microorganisms about the presence of a potential host plant [ 143 ]. For instance, putrescine and its precursor arginine attract Pseudomonas sp. and trigger a lifestyle change, promoting attachment to the root and formation of biofilm [ 144 ]. However, the best-known example is the mechanism of communication between Rhizobium and legumes. Flavonoids secreted by plants activate bacterial nod genes lead to secretion by bacteria of the nod factor. The nod factor promotes the formation of nitrogen-fixing nodules in roots [ 145 , 146 ].
Root exudates serve as a chemoattractant, by which plants “recruit” microorganisms ( Figure 1 ). Several studies showed that the composition of root exudates has an enormous effect on the composition of plant microbiome [ 147 , 148 , 149 , 150 , 151 , 152 ]. The composition of root exudates is specific for each plant species, which enables plants to attract a particular set of microbes [ 153 ]. Moreover, plants can secrete substrates that are available only for selected microbial groups or compounds that are toxic for certain groups of microorganisms in order to inhibit their growth [ 154 ]. A prominent example is the amino acid canavanine, which is present in seeds and root exudates of legumes. Canavanine is toxic to a number of soil bacteria excluding rhizobia which possess the msiA gene encoding canavanine exporter that warrants canavanine resistance [ 155 ]. Mardani-Korrani et al. [ 156 ] demonstrated that canavanine is secreted by Vicia villosa Roth, significantly decreased the diversity and changed the composition of microbial communities in soil. The presence of canavanine caused an increase in the abundance of Firmicutes and Actinobacteria and decreased the number of Proteobacteria and Acidobacteria. Another example of the selective action of compounds present in root exudates is coumarin secreted by Arabidopsis thaliana (L.) Heynh. Coumarin selectively inhibits the proliferation of some pathogenic fungi, stimulates the growth of some Pseudomonas spp. and other microbes that belong to the PGPM group, and increases the bioavailability of iron by ferric ions reduction [ 157 ]. Coumarins are used by plants to increase the bioavailability of iron. Coumarins, such as scopoletin and esculetin, enable the mobilization of Fe from minerals in acidic and alkalic soil [ 158 ]. Moreover, the depletion of Fe in soils enhances the biosynthesis and secretion of coumarins in A. thaliana [ 157 ]. It is also worth noting that fungi also produce and secrete exudates that might affect other rhizosphere microorganisms. An interesting observation was made by Toljander et al. [ 159 ], who showed that arbuscular mycorrhizal fungi (AMF) affect the composition of bacterial community through mycelia exudates. Analysis of exudates produced by Glomus sp. showed that the main components are water, low molecular weight sugars, and organic acids. Moreover, it was shown that Glomus exudates inhibited the growth of several bacterial strains including opportunistic pathogens Flavobacterium spp. and increased the abundance of bacteria belonging to Gammaproteobacteria.
Root exudates are usually secreted without energy, mostly through diffusion, ionic channel, and vesicle transport. Among several transporters involved in the secretion of exudates by roots are ABC (ATP-binding cassette) transporters which transport lipids and flavonoids [ 160 ], and anion channels are involved in secreting carbohydrates [ 161 ]. Moreover, for the secretion of root exudates transmembrane proteins aquaporins (AQPs) that are related to the membrane reflection coefficient and root hydraulic conductivity seem to be of great importance, as reviewed in [ 162 ]. Aquaporins are present in endogenous and exogenous membranes of eukaryotes and prokaryotes and are responsible for symplastic transport of not only water but also low molecular weight compounds and non-charged molecules including urea, glycerol, hydrogen peroxide, ammonium ions, and some elements, e.g., silicon and boron, as reviewed in [ 163 ]. Interestingly, it was demonstrated that during ectomycorrhiza formation the expression of fungi Laccaria bicolor aquaporins significantly increased. Moreover, fungal aquaporins exhibit high permeability for NH 3 and, therefore, it was suggested that they are involved in the transfer of this compound from fungal cytoplasm to plant [ 164 ]. Glycine max L. noduline 26 aquaglyceroporin (GmNod26) is located in the symbiosome membrane in N 2 -fixing nodules and is a transporter of ammonia. The C-terminal domain of GmNod26 interacts with the main ammonia assimilatory enzyme, i.e., glutamine synthetase, and this probably supports the effective assimilation of fixed nitrogen [ 165 , 166 ]. The potential of root exudates to determine the composition of the rhizosphere microbiome is also important for the biodegradation of various soil pollutants. Environmental pollution is a serious global challenge and needs urgent development of effective methods of remediation. An interesting observation was presented by Janczak et al. [ 167 , 168 ] who showed that the presence of plants had a positive effect on bacterial, (i.e., Arthrobacter sulfonivorans, and Serratia plymuthica ), and fungal, (i.e., Clitocybe sp. and Laccaria laccata ), ability to degrade polymers including polylactide (PLA) and poly(ethylene terephthalate) (PET). The effectiveness of biodegradation also significantly depended on the species, i.e., the presence of Salix viminalis L. (willow) enhanced the level of biodegradation more significantly that the presence of B. napus and Miscanthus x giganteus J.M.Greef, Deuter ex Hodk., Renvoize (giant miscanthus). It was suggested that root exudates probably support the growth of microorganisms and/or root exudates can activate microbial genes involved in the biodegradation of plastics, such as intra- and extra-cellular depolymerases. The breakdown of long polymers into oligo-, di-, and mono-mers enables uptake of those molecules by a bacterial cell which can be then utilized as a carbon and/or energy source [ 169 ]. Afzal et al. [ 170 ] reported that inoculation of Italian ryegrass ( Lolium multiflorum Lam.) and birdsfoot trefoil ( Lotus corniculatus L.) with alkane-degrading bacteria Pantoea ssp. and Pseudomonas sp. separately or in consortium resulted in higher biomass production by plants and bacterial consortium showed higher degradation ratio in comparison to single strain inoculants. The ability of these bacteria to degrade alkane was linked to the presence of genes encoding cytochrome p450 alkane hydroxylase (CYP153) and alkane monooxygenase ( alkB ). Enterobacter ludwigii possessing the CYP153 gene was able to degrade diesel fuel [ 171 ]. On the other hand, it was shown that the level of degradation of polycyclic aromatic hydrocarbon was reduced in the presence of ryegrass ( Lolium perenne L.) root exudates [ 172 ] which suggest that plants might differ in their potential to enhance bioremediation potential of soil microorganisms. The PGPM are able to degrade various other compounds of different origins as a means for the promotion of plant growth and development. Allelochemical compounds secreted by plants can hinder the cultivation of other plant species in cropping systems. Trichoderma harzianum SQR-T037 was shown to degrade allelochemicals secreted by cucumber roots (4-hydroxybenzoic acid, vanillic acid, ferulic acid, benzoic acid, 3-phenylpropionic acid, and cinnamic acid). The use of strains able to biodegrade allelochemicals can ameliorate allelopathic stress in continuous cropping systems [ 173 ]. Among common soil contaminants, plant protection agents are of special interest since their persistence in the agricultural soil is high, and their concentration may increase with each application. Diuron, a phenylurea herbicide, has a mean half-life of 330 days. Inoculation of soil containing diuron with fungal endophyte Neurospora intermedia leads to degradation of 99% of diuron in the soil after 3 days. Moreover, the authors reported that this strain is able to degrade other phenylurea herbicides, e.g., fenuron, monuron, isoproturon, chlorbromuron, and chlortoluron [ 174 ]. Although organophosphorus pesticides are perceived as non-persistent, they are highly toxic for a wide variety of non-target organisms, including mammals and rhizospheric microbes. Tested strains of T. harzianum and Metarhizium anisopliae showed an ability to degrade a number of organophosphorus pesticides such as diazinon, profenofos, and malathion in a temperature range of 20–45 °C [ 175 ].
3. Microbiome and Holobiont
Plants provide a multitude of ecological niches for various organisms to thrive. All these organisms including bacteria, fungi, protists, and nematodes that live on the surface and inside tissues/organs of a certain plant form the plant microbiome [ 176 ]. Although each member of the microbiome might contain genes related to the promotion of plant growth and development, the expression of those genes is dependent on the composition of the whole microbiome, on the population dynamics of potential pathogens, and on environmental conditions [ 177 ]. For example, the consortium of six bacteria ( Arthrobacter nitroguajacolicus , Bacillus cereus , Bacillus megaterium , Bacillus mojavensis , Pseudomonas azotoformans , and Pseudomonas frederiksbergensis ) was much more effective in the protection of Nicotiana attenuata Torr. ex S.Watson (coyote tobacco) against fungal pathogens Fusarium sp. and Alternaria sp. than individual members of the consortium [ 178 ]. Analysis of P. fluorescens transcriptome in response to the presence of bacteria belonging to three different genera revealed significant differences in the transcription response of P. fluorescens to different competitors [ 179 ]. Moreover, another layer of microbiome complexity is added by the presence of microbial symbionts of the members of the plant microbiome. For example, plant-associated fungi are in symbiosis with bacteria, i.e., endofungal/endohyphal bacteria [ 180 ]. Interestingly, it was shown that endohyphal bacteria Luteibacter sp. significantly increases the production of indole-3-acetic acid (IAA) in fungi Pestalotiopsis sp. However, Luteibacter sp. is not able to synthesize IAA [ 181 ]. Viruses can also interact with the plant microbiome. Dichanthelium lanuginosum (Elliott) Gould (panic grass) grows in geothermal areas in consortium with its endophytic fungus Curvularia protuberata . When the fungus is infected with Curvularia thermal tolerance virus (CThTV), soil temperature tolerance of panic grass increases from 40 °C to 65 °C [ 182 , 183 ]. Those results clearly show that other microorganisms present in soil strongly affect the PGPM potential to promote plant growth and development.
Although plants recruit a number of diverse microorganisms, they show preferences toward specific bacteria species. Analysis of seeds of medicinal plant red sage ( Salvia miltiorrhiza Bunge) collected at different locations revealed that there are no significant differences in the composition of the microbiome, whereas different plant species collected at the same location had significantly different microbiomes. This indicates that different species of plants exhibit a preference for specific groups of microorganisms [ 184 ]. Redford et al. [ 185 ] demonstrated the structure of microbial communities present on Pinus ponderosa Dougl. Ex C. Lawson needles are very similar regardless of geographic location. However, there are also examples of substantial differences in the microbiome of different cultivars of one plant species described in the literature. For example, Germida et al. [ 186 ] showed that root microbiomes of modern and older wheat cultivars were significantly different. Moreover, some microorganisms interact only with single plant species. For instance, a number of ectomycorrhizal fungi form mycorrhiza only with one species of a tree, e.g., Suillus grevillei and larch ( Larix sp.) (as reviewed in [ 187 ]). Species of Pinaceae are the only hosts for the fungal genus Rhizopogon [ 188 ]. The most known example is the highly specialized relationship between legumes and rhizobia [ 189 ]. Wicaksono et al. [ 190 ], by studying bog ecosystems, found that microbiomes of vascular plants are less diversified than those of non-vascular plants including bryophytes. Specificity between host and microbes seems to be a plastic trait modulated by the environment [ 191 ]. The mechanisms underneath the preferences of the host plant toward a specific set of microorganisms are not well understood, but recently Salas-González et al. [ 192 ] showed that mechanisms involved in the maintenance of plant mineral nutrient homeostasis also contribute to microbiome assembly.
The composition of the plant microbiome is largely dependent on the phase of plant growth and development. The mature seed is colonized by microorganisms that were associated with the mother plant, (i.e., microbes were transferred vertically), and those microorganisms are the first members of the microbiome of a newly emerging plant [ 193 ]. During germination, microbes transferred vertically have an advantage in plant colonization over the microorganisms present in the soil. During plant development, new symbiotic microorganisms transferred horizontally, (i.e., from the soil), appear [ 136 , 194 ]. Interestingly, it was demonstrated that microbes transferred vertically usually inhabit the phyllosphere, while the rhizosphere and root are colonized by the microorganisms from the soil. A study on oak ( Quercus robur L.) microbial inheritance showed that microbial composition of the phyllosphere was very similar to the composition of the embryo [ 195 ]. Sánchez-López et al. [ 196 ] showed that seed endophyte Methylobacterium sp. Cp3 was transferred via seeds across three generations of the plant Crotalaria pumila Ortega. Moreover, when Methylobacterium sp. Cp3 was inoculated to the soil at the time of C. pumila , flowering migration of bacteria from soil to seeds was observed. Moreover, the microbiome strongly differs among plant organs and tissues, i.e., the surface of the leaf is colonized by different microorganisms in comparison to the rhizosphere microbes [ 177 ]. A study on native and cultivated Agave species showed differences in bacterial taxa colonizing the rhizosphere, the phyllosphere, and the leaf and root endosphere. Interestingly, a composition of the fungal microbiome was affected mainly by the host plant biogeography [ 197 ]. Zarraonaindia et al. [ 198 ] demonstrated that below- and above-ground microbial communities of grapevine ( Vitis vinifera L.) were significantly different. Moreover, the composition of microbiomes of leaves, grapes, and flowers was more similar to the composition of soil microbiomes than to each other. Similarly, in sugarcane ( Saccharum sp.), clear differences in microbial taxa between organ types were observed. Interestingly, the microbiome composition of the young shoots formed from the underground ratoon was very similar to the microbiome of roots [ 199 ]. The phyllosphere is a hostile and dynamic environment. Microbes present on plant above-ground surfaces are subjected to irregular nutrient availability and changeable environmental conditions. The phyllosphere microbiome seems to be even more dependent on environmental conditions than the root microbiome [ 88 , 185 ]. It should be pointed out that the data regarding phyllosphere microbiomes other than leaf microbiomes is still rather limited. A study on the microbiome of apple ( Malus domestica Borkh.) flowers showed that the most popular are bacteria belonging to the extremophilic phylum Deinococcus - Thermus . Moreover, the composition of the flower microbiome was dependent on the phase of apple flower development [ 200 ].
The composition of the plant microbiome is also strongly influenced by various environmental factors, such as climate, soil properties [ 201 , 202 , 203 ], water [ 204 ], and nutrient availability [ 205 , 206 ]. The adaptation of plant metabolism to environmental change is strongly supported by rapidly changing microbial communities [ 204 ]. Analysis of the lettuce ( Lactuca sativa L.) microbiome showed that the composition of the phyllosphere microbiome is strongly dependent on the time of the year [ 207 ]. Drought is one of the most important stress factors significantly affecting not only plant growth and the yield of crops [ 208 ], but also the plant microbiome. Drought affects the microbiome directly because a low level of soil moisture inhibits the growth of several microorganisms. In drought conditions, plants recruit stress microbiomes, i.e., the most beneficial group of microbes allowing the plant to adapt to a particular set of environmental conditions. For instance, the abundance of Actinobacteria increased in drought-treated roots and the rhizosphere of 18 species belonging to Poaceae . The results suggest that although the microbiome is species-specific, drought caused a relatively conserved response in different hosts [ 209 ]. In drought stress conditions, an increase in the abundance of the rhizospheric drought stress-resistant bacteria in Oryza sativa L. was observed, mainly members of Actinobacteria and Chloroflexi, whereas the abundance of Acidobacteria and Deltaproteobacteria decreased [ 210 ]. Nelson et al. [ 211 ] reported enormous changes in microbial composition in forest soils after wildfires which occur more often due to climate change. As expected, an overall decrease in the abundance and biodiversity of bacterial and fungal communities was observed one year after the fire. Both the carbon and nitrogen cycle were found to be impaired not only by the loss of microbial taxa involved in geochemical cycles, (e.g., no expression of nifA genes in tested soils was detected), but also by the activity of viruses. The presence of plant pathogens has also a substantial effect on the microbiome ( Figure 1 ). In Gossypium hirsutum L. (cotton) infected with the pathogenic fungus Verticillium, the abundance of arbuscular mycorrhiza fungi and plant growth-promoting bacteria (PGPB) was lowered [ 212 ]. A study on strawberries ( Fragaria x ananassa Duchesne) infected with Verticillium dahliae and Macrophomina phaseolina showed that plants without symptoms of infection had a higher abundance of PGPB than plants with visible symptoms of infection. The microbiome of healthy plants includes more bacteria antagonistic or competitive towards pathogens [ 213 ]. Moreover, herbivores can shape the plant microbiome. Kong et al. [ 214 ] showed that whitefly infestation of Capsicum annuum L. (pepper) changed the overall microbiome composition. Pseudomonas spp. that are recruited by the plant to the rhizosphere microbiome increased the mortality of whitefly.
A particular part of the microbiome is the core microbiome which consists of those species of microbes that regularly and ubiquitously appear in the microbiomes of particular plant species. The concept of the core microbiome was first coined by a scientist involved in the Human Microbiome Project, with the goal to identify microbial taxa and/or genes that are shared by all or most humans [ 215 ]. Microbial taxa that are commonly found in a number of environments or host types can be assigned to a core microbiome. The most common approach to verifying whether a species belong to the core microbiome is to determine microbial groups that are shared among two or more microbiomes of a particular host in various environments [ 216 ]. For example, analysis of the B. napus rhizosphere microbiome grown in different conditions, i.e., the level of fertilization and the level of plant density, revealed that the core root microbiome of this plant is composed of microbes belonging to genera Streptomyces , Cryocola , Arthrobacter , Flavobacterium , Janthinobacterium , Serratia , Kaistobacter , Pseudomonas , Pedobacter , Agrobacterium , Burkholderia , Acidovorax, Erwinia , and Stentrophomonas [ 217 ]. Analysis of the composition of the microbiome of various plant species including A. thaliana , rice, sugarcane, grapevine, barley, and soybean has revealed that the core microbiome included microbes belonging to Pseudomonas , Agrobacterium , Methylobacterium , Sphingomonas , Erwinia , Cladosporium , Conithyrium , Resinicium , and Fusarium [ 177 ]. A special part of the core microbiome is a group of microorganisms called “hub microorganisms”. Those microorganisms strongly shape the composition of the microbiome through biotic interactions with host plants and other microbes. Microorganisms belonging to the hub species are called keystone species since they serve as mediators between the plant and members of the plant microbiome. Through the hub species, the host plant can selectively affect the composition of the associated microbiome. Removal of keystone species can result in the loss of interaction and a disturbance in the whole microbiome [ 218 ]. The analysis of the phyllosphere microbiome of A. thaliana showed that plant-parasitic oomycetes Albugo laibachii is a hub species that strongly affects the whole microbial community. As a consequence of infection with A. laibachii, high divergence between the composition of the microbiome of control and of infected plants was observed. Moreover, less variability among microbiomes of infected plants was shown [ 219 ]. In a study on corn ( Zea mays L.) hub species, the elimination of Enterobacter cloaceae from inoculum containing seven microbes resulted in a loss of a few other microbes from the microbiome. Removal of other bacteria from this system did not significantly change the microbial community which suggests that E. cloaceae functions as a keystone species [ 220 ].
A far wider concept than microbiome is the concept of holobiont which was introduced in 1991 [ 221 ]. Currently, a holobiont is defined as an organism composed of the plant host and of all the microorganisms that are associated with that particular plant. Natural selection between the plant and microbes supports the system and its stability throughout the evolution of a holobiont. In a holobiont, intricate networks of interactions between microorganisms and plant host are observed (as reviewed in [ 177 , 218 , 222 , 223 ]). All the genes present in the holobiont, i.e., plant genes and genes in the microbiome, constitute the hologenome [ 224 , 225 ]. The concept of a hologenome suggests that plants’ adaptability to the environment is determined not only by plant genes but also by genes of microorganisms. Hologenomes are responsible for shaping the phenotype of holobionts in response to a particular set of environmental conditions [ 226 ].
4. Plant Immune System in Plant–PGPM Interactions
The plant immune system plays a key role in plant–microorganism interactions. It is crucial not only for controlling pathogenic microorganisms but also for balancing the homeostasis of the microbiome and for overseeing commensal microbes [ 227 ]. The prominent role in plant–PGPM interactions play patterns recognizing receptors (PRRs), which recognize conserved microorganisms-specific molecules referred to as pathogen-/microbe-associated molecular patterns (P/MAMPs), such as flagellin, lipopolysaccharides, antibiotics, and VOCs [ 228 ]. PRRs are transmembrane multimeric protein complexes located at the plasma membranes present in all plant organs and tissues. Plant PRRs are either surface-localized receptor kinases that contain ligand-binding ectodomain and intracellular kinase domain or receptor-like proteins that do not have any intracellular signaling domain. PRRs contain various ligand-binding ectodomains that allow for the recognition of a wide range of P/MAMPs [ 228 ] and activate pattern-triggered immunity (PTI) [ 229 ]. Activation of PTI via MAMPs inhibits intensive proliferation of most microorganisms via synthesis and secretion of low-molecular-weight compounds, e.g., phytoanticipins and proteins, e.g., defensins. Moreover, plants synthesize cuticles which lead to the thickening of the cell wall [ 227 ]. Some pathogens secrete effector molecules, which disturb PTI functioning and thus allow for the infection of the plant [ 222 ]. Effector-triggered immunity (ETI) is activated by recognition of pathogen effector proteins via intracellular receptors R proteins encoded by resistance genes (R genes) [ 230 , 231 ] and via nucleotide-binding leucine-rich repeat receptors (NLR) located in the cytoplasm [ 229 ]. ETI leads to the overproduction of reactive oxygen species (ROS) and ion fluxes. As a consequence, hypersensitive response (HR) is activated which leads to apoptosis of infected plant cells that restrict the spread of infection [ 232 ].
Much less is known about the action of the plant immune system in the context of commensal microorganisms; however, there is some evidence that the plant immune system is crucial for microbiome assembly. Some strains of bacteria are able to modulate plant receptors, transcription factors, and molecules involved in the functioning of an immune system which allows for the colonization of plant tissues by other symbiotic microbes [ 233 ]. Moreover, some mechanisms used by the members of the microbiome to evade or suppress the plant immune system were also described. For example, in some symbiotic microorganisms, MAMP variants that do not activate plant immune response via PRRs have evolved. In addition, some commensal fungi are able to convert chitin into chitosan via deacetylation which induces a weaker immune response. MAMPs could be also degraded or sequestered by microbial proteases and other enzymes in order to evade recognition by PRR, as reviewed in [ 222 ]. It was also suggested that plants are able to actively ignore the presence of microbial commensals [ 234 ]. In A. thaliana, outer layers of roots low expression of PRRs and a lack of immune response in presence of pathogen- and commensal-derived MAMPs were reported. Neighboring cells harbor a high number of PRRs and show a rapid MAMP-triggered response [ 235 ].
5. Mechanisms Underneath PGPM–Plant Interactions
Plant growth-promoting microorganisms affect various aspects of plant growth and development. PGPM enhances the germination ratio [ 18 , 23 , 236 ], increases the elongation growth of the shoot and root [ 46 , 48 , 237 ], increases the biomass production [ 20 , 26 , 37 ], accelerates flowering [ 56 , 73 ], and increases the photosynthesis rate [ 27 , 42 ]. The examples of the mechanisms of action of plant growth-promoting fungi (PGPF) and bacteria (PGPB) are presented in Table 2 and Table 3 , respectively.
Examples of mechanisms of plant growth promotion by PGPF.
Examples of mechanisms of plant growth promotion by PGPB.
Several mechanisms underneath the promotion of plant growth and development by microorganisms are employed by both bacteria and fungi for example degradation of ethylene via ACC deaminase, production of phytohormones, and solubilization of various soil compounds to increase the bioavailability of nutrients ( Table 2 and Table 3 ). For sure the mechanism of greatest importance is the fixation of atmospheric nitrogen via nitrogenase, a mechanism that is specific to some specialized groups of prokaryotes (as reviewed in [ 271 , 272 ]). On the other hand, the fungi-specific mechanisms that allow for the promotion of plant growth and development includes the production of hydrophobins, swollenins, and peptaibols (please see the section Trichoderma -plant interaction—a case study, for details).
5.1. Plant Antioxidant Defence System
One of the best-known mechanisms to improve plant growth and development by PGPM is the modification of the level of antioxidants including antioxidative enzymes, e.g., superoxide dismutase (SOD), ascorbate peroxidase (APX), peroxidase (POD), catalase (CAT), glutathione reductase (GR), and non-enzymatic antioxidants, e.g., proline, glutathione (GSH), ascorbic acid, carotenoids, and phenolics [ 25 , 273 , 274 , 275 , 276 , 277 ]. Islam et al. [ 278 ] demonstrated that inoculation of Vigna radiata (L.) R. Wilczek with Bacillus cereus Pb25 increased dry biomass and yield in salt stress conditions. Salt-induced oxidative damage was reduced by enhancing the activity of plant POD, SOD, and CAT and by increasing proline content in plants. Inoculation of O. sativa with Bacillus pumilus increased the activity of rice catalase and superoxide dismutase in salt stress conditions. Moreover, inoculation of rice with B. pumilus promoted the synthesis of photosynthetic pigments and proline [ 275 ]. Accumulation of phenols and proline was observed in cucumber inoculated with arbuscular mycorrhizal fungi in salt conditions [ 279 ]. A potato co-inoculated with T. viride and plant pathogen Alternaria solani showed improved redox homeostasis via increased activity of CAT and SOD, and enhanced concentration of free phenolics. Moreover, co-inoculation with T. viride and A. solani resulted in increased H 2 O 2 production which induced the expression of plant defense genes [ 274 ]. Chen et al. [ 25 ] reported increased salt stress tolerance in maize after inoculation with B. amyloliquefaciens SQR9. Inoculated plants showed a reduced level of Na + , a higher glutathione content, a higher concentration of soluble sugars, and enhanced activities of peroxidase and catalase. B. amyloliquefaciens enhanced chlorophyll content and promoted the overall growth of inoculated plants in comparison to control plants. In addition to the well-known elements of the antioxidant system, there are also other proteins exhibiting antioxidant activity, including small cysteine-rich proteins metallothioneins (MTs). MTs act as direct antioxidants since the reduced thiol groups (-SH) can be oxidized by ROS. MTs also serve as a donor of zinc and copper to other antioxidative enzymes [ 280 , 281 ]. Inoculation of rapeseed with fungal strains isolated from forest soil showed varied expressions of B. napus metallothioneins ( BnMT1 - BnMT3 ). L. laccata inoculated plants showed significant upregulation of BnMT2 expression with a decrease in BnMT3 transcripts [ 43 ].
5.2. Phytohormones
Some bacteria are able to synthesize and secrete phytohormones and thus regulate plant growth and development. For example, B. subtills synthesizes auxins and gibberellins [ 282 ], and A. brasilense [ 283 , 284 ], Peanibacillus polymyxa [ 285 ], and P. fluorescens [ 286 ] produce cytokinins. Inoculation of S. tuberosum damaged by insect attack (beetle Leptinotarsa decemlineata ) with B. subtilis 26D led to increased concentration of zeatin-riboside but not of abscisic acid (ABA) and indole acetic acid (IAA). The inoculation of potatoes with B. subtilis increased the mass of roots [ 287 ]. In the culture of Bacillus aryabhattai abscisic acid, indole acetic acid, cytokinins, and gibberellic acids were detected. Soybean inoculated with these bacteria produced more IAA, jasmonic acid, and some gibberellic acids. Moreover, inoculated plants displayed increased tolerance to heat stress possibly due to the ABA-induced closure of stomata [ 288 ].
Although different stressors affect plant organisms in various ways, most of them lead to increased ethylene production in plants. Weak stress factors can cause small overproduction of ethylene which leads to the activation of plant stress-related genes. Long periods of stress and severe stressors cause a high level of ethylene production, which might lead to senescence, chlorosis, and organ abscission [ 136 ]. Some PGPM are able to lower the level of ethylene through secretion of ACC deaminase, i.e., the enzyme that breaks down ethylene precursor 1-aminocyclopropane-1-carboxylate (ACC). Inoculation of plants with microbes producing ACC deaminase effectively increased the plant resistance to stress caused by fungal pathogens [ 289 ], nematodes [ 290 ], and several abiotic stresses such as flooding [ 291 ], drought [ 135 ], salination [ 133 ], heavy metals [ 292 ], and toxic contaminates [ 134 ]. For example, inoculation of pearl millet seed with ACC deaminase-producing B. amyloliquefaciens increased plant growth in drought stress via increasing the level of enzymatic and non-enzymatic antioxidants [ 272 ]. Isolated from Brassica rapa L. rhizosphere bacteria belonging to Pseudomonas sp. improved biomass and yield of B. rapa . The positive effect of analyzed strains on B. rapa is possibly due to the production of IAA, ACC deaminase, and siderophores [ 95 ].
5.3. Availability of Micro- and Macronutrients
In most terrestrial ecosystems, nitrogen is the major nutrient limiting plant growth. PGPMs might increase the pool of nitrogen available for plants thorough various mechanisms. It is estimated that in nature more than 60% of fixed nitrogen is a result of biological nitrogen fixation [ 272 ]. A well-known example is the Rhizobia present in root and stem nodules of legumes that is able to reduce N 2 to ammonia. There are also other symbiotic, plant-endophytic, and free-living bacteria able to fix molecular nitrogen, including bacteria belonging to Frankia , Cyanobacteria , Azotobacter , Bacillus , and Azospirillum, as reviewed in [ 293 ]. The Co-inoculation of beans with Rhizobium phaseoli and bacteria belonging to Bacillus and Pseudomonas improved plant growth by enhancing the total content of nitrogen in plant tissues more efficiently than inoculation with R. phaseoli. Only [ 127 ]. Hungria et al. [ 130 ] reported that the co-inoculation of soybean seeds with Azospirillum and Bradyrhizobium significantly enhances the yield without any input of nitrogen fertilizers. Several lines of evidence showed that rhizobia are susceptible to drought-stress and the efficiency of N 2 -fixation dramatically declines in low-water conditions [ 294 ]. For example, co-inoculation of a common bean with Rhizobium tropici and two strains of P. polymyxa more effectively increased nitrogen content and promoted plant growth than inoculation with Rhizobium only especially in drought conditions [ 295 ].
Phosphorus is also considered as element limiting plant growth in most ecosystems. Although phosphorus is an abundant element in ecosystems, most of it is not bioavailable [ 296 ]. There is a group of microbes, phosphate solubilizing microorganisms (PSM), that are able to increase the available fraction of phosphorus for plants via solubilization and mineralization mediated by secretion of organic acids, phosphatases, protons, and exopolysaccharides [ 297 ]. Red clover ( Trifolium pratense L.) inoculated with phosphate solubilizing fungi Penicillium albidum showed a significant increase in root biomass. In soil inoculated with fungi, the phosphatase activity was 1.5-fold higher than in the non-inoculated soil [ 129 ]. In soil inoculated with Burkholderia sp., Gluconobacter sp., and Pseudomonas striata, higher activity of dehydrogenase and phosphatase and a higher level of available P were detected. Vigna unguiculata (L.) Walp. grown in inoculated soil had noticeably higher biomass and yield than plants grown in non-inoculated soil. Moreover, tested microorganisms enhance uptake not only of phosphorus but also of nitrogen [ 298 ]. Inoculation of T. aestivum with Serratia marcescens enhanced plant growth and nutrient uptake (P, N, and K) in low temperatures. The ability of tested bacteria to solubilize P decreased at low temperatures [ 299 ].
Soil minerals, such as feldspars and micas, are the most common form of potassium in soils. Up to 90–98% of soil potassium is present in a form unavailable for plants [ 300 ]. By secretion of organic acids and capsular polysaccharides, some PGPM are able to solubilize potassium rocks, e.g., bacteria belonging to Acidothiobacillus , Bacillus , Pseudomonas , Burkholderia , and Peanibacillus [ 301 ]. Ali et al. [ 302 ] reported that inoculation of potatoes with K-solubilizing B. cereus resulted in significantly improved plant growth and yield. Moreover, the content of K in potato tubers and the content of N, P, and K in leaves was higher in comparison to control plants. Inoculation of ryegrass with Mesorhizobium sp., Peanibacillus sp., and Arthrobacter sp. isolated from canola rhizosphere improved biomass and yield. The content of available K in soil was much higher and resulted in increased K content in plants [ 303 ]. Basak and Biswas [ 304 ] demonstrated that treatment of waste mica with Bacillus mucilaginosus led to the transformation of K forms into water-soluble forms. This had a positive effect on K uptake and biomass of sudan grass ( Sorghum vulgare Pers.). Similar effects were observed by Raji and Thangavelu [ 305 ], who analyzed the effect of inoculation of tomato ( Lycopersicon esculentum L.) grown in Alfisol and Vertisol soils with B. subtilis , B. cereus , Bacillus licheniformis, and Burkholderia cenocepacia . Inoculated plants showed higher K content in tissues and improved growth.
The deficiency of microelements, including copper (Cu), iron (Fe), zinc (Zn), and manganese (Mn), is also a prominent factor that negatively affects plant health. In calcareous soils (widespread in arid and semiarid regions of the world), the high content of calcium carbonate which acts as a buffer and maintains a pH above 7.5, is correlated with decreased bioavailability of Fe, Zn, and Mn [ 306 ]. Plants possess mechanisms allowing them to increase amounts of bioavailable microelements in soils. For example, to facilitate Fe acquisition, plants secrete siderophores, organic acids, and flavonoids [ 307 ], whereas the amount of bioavailable Zn is increased by the secretion of organic acids, such as propionic acid, formic acid, lactic acid, citric acid, succinic acid, malic acid, oxalic acid, and gluconic acid as well as by secretion of siderophores, as reviewed in [ 308 ]. A tomato grown in hydroponic culture with the addition of soil minerals in Cu-deficient conditions showed increased biomass and Cu uptake after inoculation with Trichoderma harzianum SQR-T037 in comparison to non-inoculated plants. Interestingly, inoculation of a tomato with T. harzianum SQR-T037 grown in an Fe-deficient hydroponic culture with the addition of solid mineral increased the Fe content in plant tissue, but the biomass of seedling was unaffected. In addition, inoculation of tomatoes grown in Zn-deficient hydroponic conditions did not increase the biomass and the concentration of Zn in plant tissues was decreased. Those observations suggest that in element-deficient conditions fungi compete with plants for nutrients [ 309 ]. Rana et al. [ 128 ] showed enhanced yield and increased concentrations of Fe, Zn, Cu, and Mn by 13–16% in rice grains after inoculation with Brevundimonas diminuta , Ochrobactrum anthropic , and Providencia sp. Wheat inoculation with Providencia sp. significantly increased yield and the content of Fe and Cu in grains was 45% higher than in control plants. Singh et al. [ 310 ] reported that endophytic bacteria isolated from wheat, such as B. subtilis , Arthrobacter sp., A. sulfonivorans , and Enterobacter hirae exhibiting plant growth-promoting traits, enhanced Fe and Zn fortification as well as yield and dry/fresh weight in pot experiment and field conditions. Moreover, a decrease in phytic acid was observed in grains of wheat plants inoculated with endophytes. A field study on common bean and wheat fortification showed that inoculation with A. brasilense and T. harzianum significantly enhanced micronutrients, i.e., Fe, Mn, and Zn content in both tested plants [ 311 ]. Enhanced content of selenium was observed in lettuce inoculated with bacteria Bacillus sp., Klebsiella sp., Acinetobacter sp., and with fungus Rhizophagus intraradices in drought stress conditions. Results showed that plants inoculated with bacteria showed higher biomass production in comparison to plants inoculated with fungus, and Klebsiella sp. was the most effective in the induction of Se accumulation in lettuce. Moreover, tested microbes increased drought stress resistance, the chlorophyll and carotenoid content, and enhanced the level of antioxidant enzymes [ 312 ]. The ability of some PGPM to increase the content of macro- and micro-elements in different parts of plants is also important for human nutrition. Microelements deficiency (especially zinc and iron) is widespread all over the world. Biofortification, i.e., approaches to enhance the nutritional value of crops, with microelements in edible parts of plants, is the most promising approach to fight against microelements deficiency, as reviewed in [ 313 , 314 , 315 ]. Therefore, PGPM seems to be of crucial importance for both food quantity, i.e., enhanced plant yield and food quality, i.e., edible parts of plants with high content of minerals.
5.4. Direct Interactions of PGPM with Plant Pathogens
PGPM might directly interact with various plant pathogens. Through various mechanisms, including the production and secretion of antimicrobial metabolites, antagonisms, mycoparasitisms, and competing for niche PGPM can enhance plant biotic stress resistance. Cabrefiga et al. [ 316 ] reported an antagonistic interaction between P. fluorescens EPS62e and Erwinia amylovora , bacteria causing fire blight disease on pear trees ( Pyrus sp.). P. fluorescens EPS62e did not produce antibiotics and required cell-to-cell contact with the pathogen in order to inhibit their growth on pear flowers and fruits. Interestingly, antagonistic activity was not shown when bacteria were grown in an Fe-rich medium, which suggested that the production of siderophores is responsible for the P. fluorescens EPS62e ability to inhibit the growth of E. amylovora . Inoculation of plants with antibiotic-producing microorganisms might lead to the suppression of various plant diseases. From the roots and rhizosphere soil of cucumber grown in soil inoculated with biocontrol agent B. subtilis, antibiotics surfactin and iturin A were extracted [ 317 ]. Bais et al. [ 318 ] showed that the biocontrol ability of B. subtilis against Pseudomonas syringae is tightly linked with the production of surfactin involved in biofilm formation on A. thaliana roots. B. subtilis mutant with a deletion in the surfactin synthase gene was unable to form biofilm and was ineffective in protection against P. syringae attack. Rhizospheric S. plymuthica HRO-C48 isolated from the rhizosphere of rapeseed produces antibiotic pyrrolnitrin and can protect plants against Verticillium wilt [ 319 ]. Kurze et al. [ 320 ] demonstrated that S. plymuthica HRO-C48 protects strawberries against fungal pathogens V. dahliae and Phytophtora cactorum . Moreover, the inoculation of strawberries with S. plymuthica promoted plant growth and improved yield. Inoculation of wheat seedlings with Trichoderma sp. resulted in an increase in plant resistance markers when plants were infected with Fusarium spp. Moreover, a decrease in IAA was observed. Biocontrol activity of Trichoderma was connected with the secretion of lytic enzymes and fungal elicitors as well as mycoparasitism [ 49 ]. An interesting observation was made by Chen et al. [ 321 ] who showed that Pseudomonas piscium isolated from wheat is able to alter histone acetylation in pathogenic Fusarium gramineareum and, therefore, reduce the fungus level of virulence and mycotoxin production by the fungus. An identified compound secreted by P. piscium, i.e., phenazine-1-carboxamide, disturbs the activity of fungal histone acetyltransferase FgGcn5, responsible for the regulation of gene expression involved not only in virulence and the growth of the mycelium, but also in asexual and sexual reproduction and stress response.
5.5. Induction of the Plant Resistance by Microbial Elicitors
Although the use of living microorganisms is a potent tool in the development of sustainable agriculture, it has also numerous constraints due to legal regulations [ 322 ]. As an alternative to the living microbes and cell wall polymers (CWP) of bacteria, fungi can be employed as elicitors [ 323 , 324 , 325 ]. Elicitors trigger the immune response of the plant via numerous mechanisms including accumulation of lignin, antimicrobial enzymes, e.g., chitinases, glucanases, phytoalexins, and proteins related to the response to the presence of pathogens, guaiacol, and ribonuclease [ 326 ]. One of the efficient elicitors is chitin and its deacetylated derivative chitosan (N-acetylglucosamine subunits are linked by (1 → 4) -β bonds). For example, chitosan led to an increase in the systemic resistance in tomatoes and an increase in the plant resistance towards Alternaria solani and Xanthomonas vesicatoria [ 327 ]. Treatment of Psammosilene tunicoides with chitosan led to an increased expression of genes encoding antioxidant enzymes and transcription factors controlling stress-response genes. Moreover, the content of secondary metabolites terpenoid saponins increased [ 328 ]. The cost-effective and efficient source of elicitors are fungi belonging both to Ascomycota and Basidiomycota. Research by Nowak et al. [ 326 ] showed that (1 → 3) -α-D-glucooligosaccharides (GOS) obtained by hydrolysis of (1→3)- α- D -glucan from Laetiporus sulphureus induced the growth of wheat seedlings. Moreover, GOS caused the increase in the activity of CAT and APX, in the activity of chitinase, and higher activity of enzymes activating phenylpropanoid-producing pathways. Laminarin, a polysaccharide, consists of β-(1-3)-glucan with β-(1-6)-linkages of 20–25 units isolated from brown algae, which is an example of elicitor belonging to β-glucans. Treatment of grapevine-cultured cells with laminarin led to calcium influx, an oxidative burst, and the induction of pathogen-related genes. Laminarin by the induction of plant resistance indirectly contributes to the reduction of the growth of B. cinerea and Plasmopara viticola on grapevine plants [ 329 ].
6. The Stringent Response in Plant–Microorganism Interactions
Among several plant mechanisms regulating growth, development, and the response to environmental factors, the stringent response is of particular interest. The stringent response was first discovered in Escherichia coli in response to amino acid starvation [ 330 ]. The hallmark of the stringent response is the accumulation of atypical regulatory nucleotides guanosine tetra- (ppGpp) and pentaphosphates (pppGpp) called alarmones that are responsible for pleiotropic adaptation to nutrient deficiency and stress factors [ 331 , 332 ]. Moreover, the bacterial stringent response through regulation of quorum sensing indirectly affects the formation of microcolonies and the development and functioning of biofilm [ 333 ]. Alarmones are synthesized from ATP/GTP or GDP by enzymes possessing active synthetase domain (SYNTH) and are hydrolyzed to GTP/GDP and pyrophosphate by enzymes containing active hydrolytic domain (HD). Gram-negative bacteria usually possess two separate enzymes, i.e., alarmones synthetase RelA and alarmones hydrolase SpoT. Gram-positive bacteria usually possess one bifunctional Rel protein [ 334 , 335 ]. RelA and SpoT belong to the long RSH (RelA/SpoT homologue), i.e., enzymes possessing HD and SYNTH domains. In bacteria, there are also short RSH, i.e., enzymes containing either SYNTH domain, small alarmone synthases (SAS) or HD domain, or small alarmone hydrolases (SAH), whereas in animals, to date only small alarmone hydrolases were identified (Mesh1—metazoan SpoT homologue) [ 336 ]. Alarmones regulate transcription, translation, and DNA replication, and trigger metabolical and physiological changes in response to unfavorable environmental conditions. Upon accumulation of alarmones, bacteria change their lifestyle from growth and proliferation to survival mode [ 335 ]. Sanchez-Vazquez et al. [ 337 ] demonstrated that in E. coli, the elevated (p)ppGpp level affected the expression of 757 genes five minutes after the induction of the stringent response and after another five minutes the expression of 1 224 genes was affected. Activation of the stringent response can be triggered by a deficiency of amino acids [ 330 ], fatty acids [ 338 , 339 ], iron [ 340 ], carbon [ 341 ], nitrogen [ 342 ], phosphorus [ 343 ], and by other types of stress, e.g., increased temperature [ 344 , 345 ], cell wall antibiotics, ethanol and acid treatments, superoxide stress [ 346 ], and alkaline shock [ 347 ].
The presence of (p)ppGpp in photosynthetic Eucaryota was confirmed in the algae Chlamydomonas reinharditi [ 348 ]. In higher plants, RSH genes were identified for the first time in A. thaliana [ 349 ] and subsequently in other plant species, e.g., Nicotiana tabacum L. [ 350 ], rice [ 351 ], Ipomoea nil L. Roth [ 352 ], Sueda japonica Forssk. ex J.F. Gmel. [ 353 ], pepper [ 354 ], and non-vascular plants, e.g., Physcomitrella patens (Hedw.) Bruch and Schimp [ 355 ]. Plant RSH proteins are divided into three groups, i.e., RSH1, RSH2/3, and CRSH. All identified plant RSH proteins belong to the long RSH, i.e., possess both HD and SYNTH domains. In model plants, the A. thaliana SYNTH domain of RSH1 (AtRSH1) is Inactive due to the substitution of conserved glycine residue required for its activity. AtCRSH does not possess a functional hydrolase domain and in AtRSH2/3, both SYNTH and HD domains are active [ 356 ]. In addition to HD and SYNTH domains, RSH possess chloroplast transit peptide [ 357 ], TGS (RSH1, RSH2/3), and ACT domains (RSH1) which were proposed to act as regulatory- or ligand-binding domains [ 358 ]. CRSH are the only proteins involved in alarmones metabolism that possess two EF hand motifs and are activated by Ca 2+ ions [ 359 ]. The domain structure of RSH proteins is highly conserved across plant species. It is now widely accepted that in plants, the place of alarmones action are chloroplasts [ 360 , 361 , 362 , 363 ]. Alarmones act as regulators of plant development and growth, i.e., (p)ppGpp coordinate micro- and macro-elements redistribution during senescent [ 364 ]. They modulate the level of phytohormones [ 365 ], lipids [ 366 , 367 ], and secondary metabolites [ 368 ] in chloroplasts. Moreover, alarmones promote the replication of plastidial DNA [ 364 ]. Plant RSH genes are differently expressed in presence of biotic and abiotic stress factors, which suggest that alarmones contribute to plant response in a number of stress factors, i.e., oxidative stress [ 369 ], nitrogen starvation [ 369 , 370 ], wounding, salination, drought, UV radiation, heat shock, heavy metals, and abrupt change from light to darkness [ 371 ]. Masuda et al. [ 372 ] showed that RSH probably play a role in plant reproduction, as the AtCRSH knockdown mutant produced smaller siliques and a lower number of seeds. An interesting observation was made by Ono et al. [ 373 ], who demonstrated an increased (p)ppGpp concentration in chloroplast upon a light-to-dark transition. The alarmones accumulation was due to higher activity of CRSH caused by elevated levels of Ca 2+ in chloroplasts. As a consequence, the expression of plastidial genes is adapted to darkness. Interestingly, it was demonstrated that the accumulation of alarmones in plants leads to a decreased expression of genes involved in the defense system, which can lead to higher susceptibility to infections [ 365 ].
Plants are probably able to manipulate the level of alarmones synthesis in members of their own microbiome but also in pathogenic bacteria. Through modulation of (p)ppGpp production in bacteria, plants may be able to decrease virulence and inhibit the growth of pathogens. Nowicki et al. [ 374 ] demonstrated the activation of stringent response in E. coli cells by plant secondary metabolites isothiocyanates (ITC). ITC-induced stringent response in E. coli led to growth inhibition, disturbed transcription, and DNA replication. The induction of the E. coli stringent response may be the result of a direct interaction of ITC with cellular proteins. That idea is plausible since sulforaphane (one of the ITCs) inhibits the growth of numerous bacteria and recently potential target proteins of ITC were identified [ 375 ]. Mwita et al. [ 376 ] showed that the expression of SasA (short alarmone synthase) of PGPB Bacillus atrophaeus UCMB-5137 is considerably upregulated by maize root exudates. Further implications of observation on these bacteria metabolisms need, however, further evaluation. On the other hand, inoculation of plants with PGPB can affect plant RSH gene expression; however, the data are scarce. Dąbrowska et al. [ 377 ] demonstrated that B. napus inoculated with S. plymuthica and Serratia liquefaciens exhibited elevated mRNA levels of BnRSH1 in cotyledons and roots, whereas inoculation with Massilia timonae increased BnRSH1 expression only in roots. Moreover, S. plymuthica seemed to affect also the expression of BnRSH2 and BnRSH3 in cotyledons and roots. The relative transcript level of BnCRSH was elevated in cotyledons in presence of S. plymuthica and S. liquefaciens . Inoculation of canola grown in salt-stress conditions with endophytic Pseudomonas stutzeri ISE12 significantly increased mRNA levels of BnRSH1 and BnRSH3 in roots in comparison to non-inoculated plants grown in salt stress [ 34 ]. Moreover, Givens et al. [ 350 ] observed a 10-fold increase in RSH2 protein level in N. tabacum after infecting the plant with the bacterial pathogen Erwinia carotovora .
Microbes might directly and/or indirectly activate the stringent response in other microorganisms. A study on the effect of the pathogenic fungus Rhizoctonia solani on the rhizosphere microbiome of sugar beet ( Beta vulgaris L.) showed that in several rhizobacteria, the expression of genes involved in (p)ppGpp metabolism is upregulated. It is not clear whether the activation of the stringent response in bacteria present in the rhizosphere is triggered directly by oxalic and phenylacetic acids secreted by R. solani or indirectly by signaling molecules in root exudates [ 378 ]. Interestingly, the relA and relA / spoT mutants of Pseudomonas sp. DF41 and Pseudomonas chlororaphis PA23, showed increased antifungal activity against Sclerotinia sclerotiorum . All mutants produced an increased level of antifungal antibiotic pyrrolnitrin, lipase, and protease in comparison to wild-type bacteria. The lack of (p)ppGpp led also to reduced transcription of rpoS [ 379 , 380 ]. Selin et al. [ 381 ] showed that expression of rsmZ , rsmE, and rsmA, i.e., elements involved in the regulation of several processes such as virulence, motility, and biocontrol abilities, was regulated via the stringent response in P. fluorescens , a PGPB inhibiting the growth of a number of pathogenic fungi including S. sclerotiorum . Takeuchi et al. [ 382 ] reported that a mutant of P. fluorescens CHA0 lacking the ability to synthesize alarmones, produced significantly fewer antibiotics and had lower biocontrol activity against P. ultimum . Moreover, the ability of the tested mutant to colonize cucumber roots, both in the presence and absence of P. ultimum , was reduced possibly due to impaired motility. The stringent response was found to strongly influence the production of antibiotics also in Streptomyces which are common members of the plant microbiome. Ochi [ 383 ] reported that rel mutant of Streptomyces antibioticus showed induction of phenoxazinone synthase, an enzyme involved in the production of actinomycin. Interestingly, this mutation did not affect the activity of another enzyme participating in the biosynthesis of actinomycin, i.e., kynurenine formamidase. Moreover, RSH genes regulated the morphological and physiological differentiation of Streptomyces clavuligerus ; the lack of (p)ppGpp affected spore formation [ 384 ].
Recent studies showed that the stringent response may play a crucial role in the interaction between legumes and rhizobia. At the beginning of the interaction, legumes produce antimicrobial compounds that lead to nutritional, osmotic, and oxidative stress in rhizobia [ 385 , 386 , 387 ]. Based on the literature, it might be hypothesized that in order to survive these unfavorable conditions, rhizobia activates the stringent response. Soybean inoculated with the rsh knockout Bradyrhizobium diazoefficiens formed smaller nodules than those present in plants inoculated with wild-type B. diazoefficiens . Moreover, the biomass of plants inoculated with mutant bacteria was smaller in comparison to wild-type bacteria, but still higher than the biomass of non-inoculated plants. Those results suggest that the lack of alarmone signaling altered nodulation and, as a consequence, decreased N 2 fixation. Interestingly, in plants co-inoculated with wild-type bacteria and the rsh mutant in equal proportion, only 26% of nodules were infected by mutant bacteria. This observation strongly suggests that the stringent response is crucial to win the competition for a niche with other rhizobia [ 388 ]. In the early phase of nodulation, establishing a symbiotic relationship between rhizobia and legumes requires plant-bacteria signaling that allows the recognition of bacteria by the host. It was shown that relA mutant of Sinorhizobium meliloti failed to form nodules on M. sativa due to disturbed stringent response, but remarkably the nodulation of Medicago truncatula Gaertn. was successful. The N 2 fixation capacity of mutant bacteria was reduced in comparison to the wild type. It is not clear why there is a difference in nodulation between those two Medicago species; however, it might be hypothesized that different plants influenced bacterial stringent response at different stages of invasion [ 389 ]. Another study also found that S. meliloti mutant unable to synthesize (p)ppGpp did not establish a symbiotic relationship with M. sativa . The relA mutant of S. meliloti produced more succinoglycan, an exopolysaccharide needed for colonization of the host, than the wild-type bacteria [ 390 ]. The inactivation of relA in Rhizobium etli caused nodules on P. vulgaris form, but the level of nitrogen fixation was significantly reduced. Mutants showed significantly lower expression of raiI and cinI genes which encode regulators of quorum sensing in rhizobia [ 391 ]. Calderón-Flores et al. [ 392 ] reported that in P. vulgaris inoculated with R. etli, the rsh mutant nodulation and nitrogen fixation were disturbed. Qiu et al. [ 393 ] demonstrated that the addition of water-soluble humic materials into inoculum containing Sinorhizobium fredii significantly downregulated RSH expression, increased survivability of bacteria in soil, and promoted rhizoplane colonization of Glycine max (L.) Merr.
The stringent response is also a significant mechanism contributing to the virulence of various plant pathogens. A study on E. amylovora showed that cells of relA and relA / spoT mutants were significantly longer both in nutrient-rich and nutrient-limited conditions in comparison to the wild type. Moreover, it was demonstrated that the proliferation rate of relA / spoT mutant in pear fruits was 1000 times slower than the proliferation rate of the wild type. In minimal medium double, the relA / spoT mutant was unable to grow. Small-sized cells are more resistant to stress, and bigger relA / spoT knockouts are unable to survive on plant surfaces. It seems that (p)ppGpp are important regulators of cell growth in E. amylovora during plant infection [ 394 ]. A similar observation was made for P. syringae , one of the most common plant pathogens. P. syringae single ( relA ) and double ( relA / spoT ) knockout mutants grown on nutrient-rich medium were slightly bigger in comparison to the wild-type bacteria. An in vivo study demonstrated that relA / spoT mutants, even though they had bigger cells, were unable to survive on the surface of a tomato leaf. These findings suggest that the stringent response is a crucial element of plant surface colonization [ 395 , 396 ]. A great induction of relA and spoT genes in gram-negative bacterium Pectobacterium atrosepticum , able to degrade plant cell walls, was observed when bacteria were grown in high-density culture in carbon-deficient media [ 397 , 398 ]. Zhang et al. [ 399 ] reported that the Xanthomonas citri double knockout spoT / relA mutants showed a significant decrease in pathogenicity and inhibited growth in planta. Interestingly, the deletion of only the main alarmones synthase in the X. citri relA did not affect the virulence of the bacterium.
Several lines of evidence showed that the stringent response plays a crucial role in the adaptation of bacterial growth and metabolism to nutrient-limited conditions. The mentioned studies above show the importance of the stringent response for the establishment of plant–PGPM interactions and proper functioning of the plant microbiome. We hypothesize that the stringent response probably allows members of the microbiome to survive in unfavorable conditions during the first stages of interaction establishment. The data about the possible role of alarmones in an intricate network of interactions between plants and microorganisms, among members of microbiomes, and between PGPM and plant pathogens are rather limited. The possible crucial role of the plant and the microbial stringent response in the functioning of microbiomes is an exciting but rather overlooked research area that needs further experiments.
7. Trichoderma –Plant Interaction—A Case Study
Among several other microbes belonging to the PGPM group, fungi from the genus Trichoderma are of great interest. Adaptability to unfavorable environmental conditions and the ability to utilize many different substrates as nutrients determine the ubiquitous occurrence of fungi belonging to Trichoderma in soils. It is estimated that one gram of soil contains 10–10 3 CFU (colony-forming unit) of fungi belonging to Trichoderma [ 400 , 401 ]. It was estimated that there are around 438 species in the genus Trichoderma , grouped into 10 phylogenetic lineages: Brevicompactum, Deliquescens, Harzianum, Hypocreanum, Longibrachiatum, Polysporum, Psychrophilum, Semiorbis, Stromaticum, and Viride [ 402 ].
Through interaction with plant fungi belonging to Trichoderma gains, a convenient niche for growth and development since root exudates are a rich source of carbon and other nutrients. The presence of fungi belonging to Trichoderma enhances growth and increases yield [ 403 , 404 ], improves uptake of nutrients by plants [ 405 , 406 ], and leads to a higher vigor and germination ratio of seeds [ 407 , 408 ]. In addition, fungi belonging to Trichoderma increase the level of photosynthesis [ 409 ], the level of amino acid synthesis [ 410 ], the level of transpiration [ 411 ], and the water content in tissues in drought conditions [ 412 ]. Fungi from the genus Trichoderma can colonize the roots of mono- [ 56 , 57 , 413 ] and di-cots [ 58 , 131 , 132 ] and when plants are grown in acidic soils [ 414 ], alkaline soils [ 415 , 416 ], and soils contaminated with heavy metals [ 417 , 418 ]. Trichoderma are potential symbionts of non-mycorrhizal plants belonging to Brassicaceae [ 59 ], Chenopodiaceae [ 302 ], Caryophyllaceae [ 419 ], Polygonaceae [ 420 ], and others. Recently, marine isolates of Trichoderma have been identified [ 421 , 422 , 423 , 424 , 425 ], which have the potential to serve as plant growth-promoting fungi for plants grown in saline soils [ 426 ]. Several mechanisms have been shown to contribute to the promotion of plant growth and development by fungi belonging to Trichoderma . Colonization of plants by Trichoderma changes host proteome [ 413 ] and secretome [ 427 ], affecting the level of synthesis of phytohormones [ 428 ] in soluble sugars [ 409 ] and phenolic compounds [ 173 , 429 ]. The inoculation of A. thaliana seedlings with Trichoderma virens and Trichoderma atroviride increased biomass production and promoted lateral root growth. Mutations in plant genes involved in auxin transport and signaling, i.e., AUX1, BIG, EIR1 , and AXR1 caused reduced stimulation of root growth and development by tested Trichoderma isolates [ 92 ]. Fungi belonging to Trichoderma compete with pathogens for ecological niches and nutrients, which efficiently limits the growth of pathogens. Secreting antibiotics, siderophores, a range of volatile and non-volatile metabolites (n-alkanes, cyclohexane, cyclopentane, esters, alcohols, sulfur-containing compounds, pyrane, and benzene derivatives), and through mycoparasitism fungi belonging to Trichoderma, protect plants against various pathogens, such as R. solani [ 430 , 431 , 432 ], Rhizopus oryzae [ 433 ], Fusarium spp. [ 434 , 435 ], Alternaria alternate [ 436 ], S. sclerotiorum [ 432 , 437 ], Botrytis cinerea [ 438 , 439 ], Pythium spp. [ 433 ], and Ustilago maydis [ 440 ]. Several secondary metabolites produced by fungi belonging to Trichoderma peptaibols seem to be of great importance for Trichoderma biocontrol activity. Peptaibols are amphipathic polypeptides composed of 5–10 amino acids with molecular masses between 500 and 2200 Da. These non-ribosomally synthesized polypeptides contain not only typical amino acids but also non-proteinogenic amino acids and α-aminoisobutyric acid. Peptaibols are synthesized not only by fungi belonging to Trichoderma but also by other soil-born fungi as well as by plant-pathogen fungi [ 441 , 442 ]. Several lines of evidence have confirmed that peptaibols exhibit antibacterial and antifungal properties. Trichoderma pseudokoningii produces trichokonin VI that induces apoptotic cell death in F. oxysporum [ 443 ]. Trichokonins A produced by Trichoderma longibrachiatum damages the cell membrane of Gram-negative pathogenic bacteria Xanthomonas oryzae pv. Oryzae , leading to a significant reduction of the pathogenicity of these bacteria [ 444 ]. The same inhibitory effect was observed for several other peptaibols produced by various Trichoderma species against a range of plant pathogens, e.g., B. cinerea [ 445 ], Septoria tritici [ 446 ], A. solani , and R. solani [ 447 ]. Moreover, it was also demonstrated that peptaibols can act against viruses. Luo et al. [ 448 ] showed that trichokonins isolated from T. pseudokoningii induces resistance of tobacco against the tobacco mosaic virus probably via induction of reactive oxygen species and phenolic compound production. Peptaibols isolated from T. virens might act as elicitors and induce a defense response in cucumber against pathogenic bacteria Pseudomonas syringae pv. lachrymans via up-regulation of hydroxyperoxide lyase, phenylalanine ammonia lyase, and peroxidase gene expression. In addition, T. virens mutant tex1 lacking one of the non-ribosomal peptide synthetases was less effective in the inhibition of P. syringae pv. lachrymans growth [ 247 ]. It should be pointed out that high concentrations of peptaibols might have a negative impact on the growth of plants, as shown for peptaibols produces by Trichoderma reesei and their negative effect on A. thaliana . However, peptaibols at lower concentrations are still sufficient to inhibit the growth of plant pathogens with no adverse effect on plant growth [ 449 ].
Moreover, some strains of Trichoderma can induce ISR (induced systemic resistance) and/or SAR (systemic acquired resistance) in the host plant through the secretion of fungal elicitors. Shoresh et al. [ 450 ] demonstrated that the treatment of cucumber with T. asperellum T203 activated ISR via the JA/ethylene signaling pathway. Inoculated plants were more resistant to P. syringae than non-inoculated control plants. Another study showed that soil inoculation with T. harzianum enhanced tomato defense against root-knot nematode ( Meloidogyne incognita ) by SAR activation and increased ethylene synthesis [ 132 ]. Inoculation of canola with T. harzianum TH12 triggered SAR and ISR defense mechanisms and decreased the severity of disease symptoms caused by S. sclerotiorum [ 132 ]. Changes in host cells caused by inoculation with Trichoderma are also visible in tissues distant from the penetration site. Yedidia et al. [ 451 ] demonstrated that the cell walls of cucumber root epidermis and cortical cells after T. harzianum inoculation were strengthened also beyond the penetration site. The activity of plant peroxidases and chitinases was upregulated by the presence of a fungus both in roots and leaves. These findings are in agreement with the fact that microbial invasion of host plant cells induces systemic resistance mechanisms. Huang et al. [ 452 ] reported that inoculation of cucumber with T. harzianum SQR-T37 significantly promoted growth as well as suppressed the damping off disease caused by R. solani . It was shown that the main mechanism of biocontrol was mycoparasitism. Between the pathogen and T. harzianum SQR-T37, a direct interaction was observed. The hyphae of T. harzianum were densely coiled, and hooks and appressorium-like bodies were formed. As a consequence, the cell walls of R. solani were broken and leakage of cytoplasm was noted.
Fungi belonging to Trichoderma are able to colonize plants due to the mechanisms allowing the recognition and the adhesion to the surface of the root ( Figure 2 ). Moreover, these fungi are able to penetrate the root tissues and suppress the plant’s immune system in order to avoid strong responses from the host, as reviewed in [ 453 , 454 ]. The plant’s immune system recognizes Trichoderma MAMPs, including swolenin [ 250 ], alamethicin [ 455 ], xylanase [ 456 ], cellulases [ 457 ], and polygalacturonase [ 458 ]. Plant responses to MAMPs are quick and transitional in the early stages, they involve ion level fluctuation, overproduction of ROS, nitric oxide, and ethylene [ 459 ]. Later stages involve the production of callus wall and antifungal compounds and, as a consequence, further penetration of the plant by hyphae is stopped [ 429 , 460 ]. It was demonstrated that salicylic acid has a particular role in callus synthesis during the colonization of plants by fungi. In a A. thaliana sid2 (salicylic acid induction-deficient2) mutant with a disturbed SA signalization, T. harzianum penetrated root vascular tissues whereas in wild-type plants, penetration of plants by fungi were restricted to outer root layers [ 460 ]. Inoculation of Z. mays with T. virens caused the reduction of plant secretome by 36%, which deprives plants of essential signaling molecules and proteins crucial for proper plant growth and development. At the same time, a fungus secretes similar compounds which do not activate the plant’s immune system [ 427 ]. The ability to colonize plant tissues by Trichoderma is tightly linked with the fungal capacity to tolerate secreted plants’ antimicrobial compounds [ 459 ]. An ABC transporter system is crucial for the resistance of Trichoderma to antifungal compounds secreted by plant pathogens. Deletion in the Taabc2 gene in T. atrioviride caused the loss of the ability of the fungus to protect the tomato against P. ultimum and R. solani attacks [ 461 ]. Another mechanism exploited by fungi belonging to Trichoderma is directly decreasing the synthesis of the antifungal compound. For example, Masunaka et al. [ 462 ] reported that inoculation of Lotus japonicus L. with Trichoderma koningi down-regulated the production of isoflavonoid vesitol, i.e., the main phytoalexin produced by lotus species. Fungi belonging to Trichoderma were found proficient in the degradation of allelochemicals secreted by plants which exhibit fungitoxicity to a number of fungi [ 173 ].

Colonization of root by a fungus belonging to Trichoderma . Adhesion and protection of hyphae are mediated by the layer of hydrophobins, whereas lytic enzymes enable penetration of the epidermis. Swollenins facilitate penetration of apoplast through an expansion-like effect on plant cell walls. Recognition of Trichoderma -derived MAMP molecules (swollenins, hydrophobins, cellulolytic enzymes, and chitin) triggers plant responses to infection, i.e., synthesis of antimicrobial compounds (defensins and phytoanticipins), synthesis of the callose wall in order to physically inhibit further penetration, and overproduction of ROS and possibly also alarmones. See text for more details.
Adhesion of fungi belonging to Trichoderma to the surface of plants is mediated by hydrophobins, i.e., small, cysteine-rich hydrophobic proteins ( Figure 2 ). Hydrophobins are synthesized by filamentous fungi. The amino acid sequence of hydrophobins is highly evolutionarily conserved. Hydrophobins are classified into two classes based on the arrangement of cysteine residues, differences in solubility, and physical properties. Hydrophobins form amphipathic monolayers at hydrophobic-hydrophilic interfaces. Those proteins are involved in the formation of aerial hyphae, fruiting bodies, and spores as reviewed in [ 463 , 464 ]. It was shown that class I hydrophobins produced by T. asperellum enabled adhesion to cucumber roots. The authors hypothesized that hydrophobins protect hyphae against antimicrobial compounds were secreted by the host during colonization [ 244 ]. Inoculation of tomato and cucumber with a T. harzianum mutant with a deletion in the gene encoding hydrophobin showed that the mutant fungi were able to colonize the roots of both plants; however, the lateral roots were significantly shorter than those present in plants inoculated with the wild-type fungus [ 245 ]. Further penetration of roots by hyphae is possible due to fungal proteolytic enzymes, e.g., aspartyl protease (PapA) [ 465 ], and cellulolytic enzymes, e.g., endopolygalacturonase (ThPG1) [ 458 ], and arabinofuranosidases (Abf1, Abf2) [ 465 ] that allow for degradation of the plant cell wall. Another important element allowing Trichoderma for tissue penetration are swolenins ( Figure 2 ), i.e., proteins possessing a cellulose-binding domain (CBD), similar to plant proteins—expansins. Swollenins disrupt the structure of the cellulose which results in changes to the plant cell wall architecture and the expansion of intercellular space [ 466 ]. Swollenins facilitate penetration of apoplast by the hyphae and give fungi an advantage during the competition for the niche with other microbes. Brotman et al. [ 250 ] reported that T. asperellum overexpressing swollenin showed a significantly improved ability to colonize cucumber roots, whereas swolenin knockout mutants showed a reduced ability to colonize roots. Moreover, it was found that the CBD domain acts as the MAMP, and can induce plant defense against B. cinerae and P. syringae . Similarly, T. atroviride overexpressing the swolenin-coding gene Taswo1 improved the colonization rate and enhanced the growth of tomatoes and peppers. Moreover, the induction of the plant immune system was stronger by mutants overexpressing swollenins than by knockouts and wild-type fungi [ 467 ].
Sucrose plays important role in plant colonization by Trichoderma . As demonstrated by Macías-Rodríguez et al. [ 468 ], the concentration of carbohydrates, mainly arabinose, xylose, myo-inositol, fructose, and glucose in root exudates of L. esculentum is higher before colonization by T. atrioviride . Colonization of the root by T. atrioviride changed the exudation pattern and sucrose became a major component of exudates. Root-derived sucrose specifically enables better growth of fungi belonging to Trichoderma, since AMF fungi prefer glucose and fructose as a source of carbon. Analysis of the proteome of maize inoculated with T. harzianum T22 showed that 40 proteins involved in carbohydrate/starch metabolism were upregulated and 13 proteins were downregulated, which suggests that T. harzianum is able to modulate carbohydrate metabolism in colonized roots [ 413 ]. T. virens was shown to produce invertase that hydrolyses plant-derived sucrose. The sucrolytic activity of fungal cells is crucial for root colonization but also to increase the photosynthetic rate in maize leaves [ 409 ].
Funding Statement
This research received no external funding.
Author Contributions
Conceptualization, G.B.D.; investigation, M.A. and A.M.-A.; data curation, M.A.; writing—original draft preparation, M.A. and G.B.D.; writing—review and editing, G.B.D. and A.M.-A.; supervision, G.B.D. and A.M.-A.; funding acquisition G.B.D. and A.M.-A. All authors have read and agreed to the published version of the manuscript.
Conflicts of Interest
The authors declare no conflict of interest.
Publisher’s Note: MDPI stays neutral with regard to jurisdictional claims in published maps and institutional affiliations.

Sustainable Agriculture Research & Education Program
- What is Sustainable Agriculture?
The goal of sustainable agriculture is to meet society’s food and textile needs in the present without compromising the ability of future generations to meet their own needs.
Practitioners of sustainable agriculture seek to integrate three main objectives into their work: a healthy environment, economic profitability, and social and economic equity. Every person involved in the food system—growers, food processors, distributors, retailers, consumers, and waste managers—can play a role in ensuring a sustainable agricultural system.
There are many practices commonly used by people working in sustainable agriculture and sustainable food systems. Growers may use methods to promote soil health , minimize water use , and lower pollution levels on the farm. Consumers and retailers concerned with sustainability can look for “ values-based ” foods that are grown using methods promoting farmworker wellbeing , that are environmentally friendly , or that strengthen the local economy. And researchers in sustainable agriculture often cross disciplinary lines with their work: combining biology, economics, engineering, chemistry, community development, and many others. However, sustainable agriculture is more than a collection of practices. It is also process of negotiation: a push and pull between the sometimes competing interests of an individual farmer or of people in a community as they work to solve complex problems about how we grow our food and fiber.
Topics in sustainable agriculture
- Addressing Food Insecurity
- Agritourism
- Agroforestry
- Conservation Tillage
- Controlled Environment Agriculture (CEA)
- Cooperatives
- Cover Crops
- Dairy Waste Management
- Direct Marketing
- Energy Efficiency & Conservation
- Food and Agricultural Employment
- Food Labeling/Certifications
- Food Waste Management
- Genetically Modified Crops
- Global Sustainable Sourcing of Commodities
- Institutional Sustainable Food Procurement
- Biologically Integrated Farming Systems
- Integrated Pest Management (IPM)
- Nutrition & Food Systems Education
- Organic Farming
- Precision Agriculture (SSM)
- Soil Nutrient Management
- Postharvest Management Practices
- Technological Innovation in Agriculture
- Urban Agriculture
- Value-Based Supply Chains
- Water Use Efficiency
- Water Quality Management
- Zero-Emissions Freight Transport
Directory of UC Programs in Sustainable Agriculture
This directory is a catalog of UC's programmatic activities in sustainable agriculture and food systems. All programs are sorted by activities and topic areas.
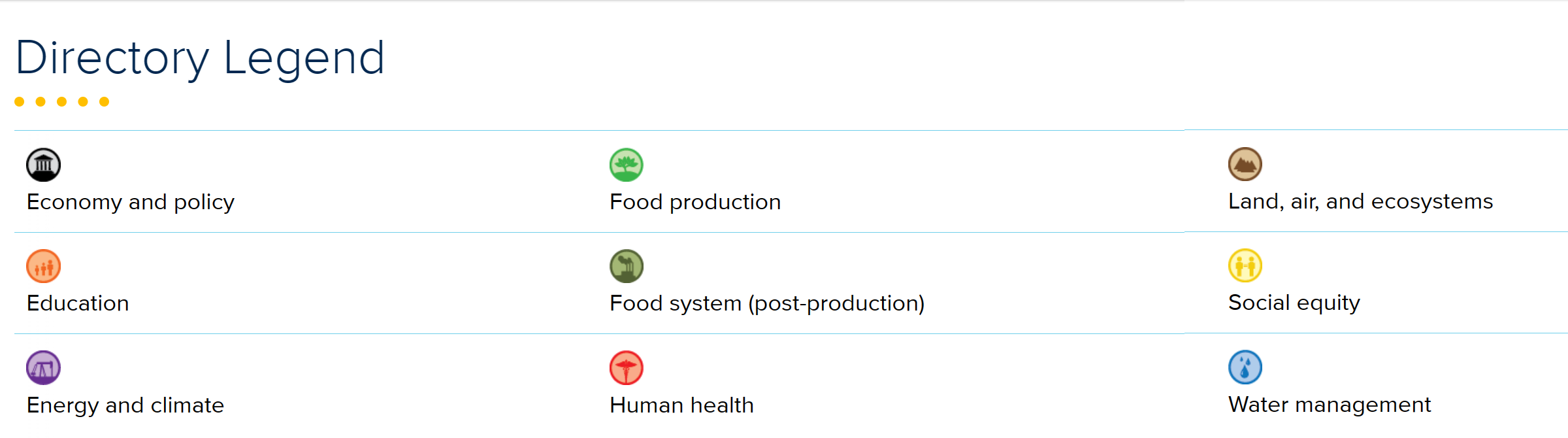
The Philosophy & Practices of Sustainable Agriculture
Agriculture has changed dramatically, especially since the end of World War II. Food and fiber productivity soared due to new technologies, mechanization, increased chemical use, specialization and government policies that favored maximizing production. These changes allowed fewer farmers with reduced labor demands to produce the majority of the food and fiber in the U.S.
Although these changes have had many positive effects and reduced many risks in farming, there have also been significant costs. Prominent among these are topsoil depletion, groundwater contamination, the decline of family farms, continued neglect of the living and working conditions for farm laborers, increasing costs of production, and the disintegration of economic and social conditions in rural communities.
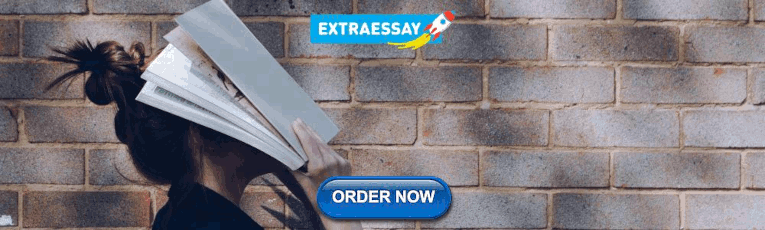
Potential Costs of Modern Agricultural Techniques
A growing movement has emerged during the past two decades to question the role of the agricultural establishment in promoting practices that contribute to these social problems. Today this movement for sustainable agriculture is garnering increasing support and acceptance within mainstream agriculture. Not only does sustainable agriculture address many environmental and social concerns, but it offers innovative and economically viable opportunities for growers, laborers, consumers, policymakers and many others in the entire food system.
This page is an effort to identify the ideas, practices and policies that constitute our concept of sustainable agriculture. We do so for two reasons: 1) to clarify the research agenda and priorities of our program, and 2) to suggest to others practical steps that may be appropriate for them in moving toward sustainable agriculture. Because the concept of sustainable agriculture is still evolving, we intend this page not as a definitive or final statement, but as an invitation to continue the dialogue
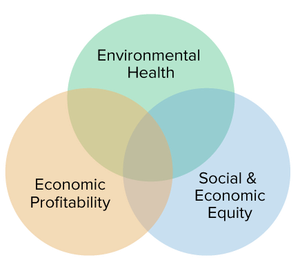
Despite the diversity of people and perspectives, the following themes commonly weave through definitions of sustainable agriculture:
Sustainability rests on the principle that we must meet the needs of the present without compromising the ability of future generations to meet their own needs. Therefore, stewardship of both natural and human resources is of prime importance. Stewardship of human resources includes consideration of social responsibilities such as working and living conditions of laborers, the needs of rural communities, and consumer health and safety both in the present and the future. Stewardship of land and natural resources involves maintaining or enhancing this vital resource base for the long term.
A systems perspective is essential to understanding sustainability. The system is envisioned in its broadest sense, from the individual farm, to the local ecosystem, and to communities affected by this farming system both locally and globally. An emphasis on the system allows a larger and more thorough view of the consequences of farming practices on both human communities and the environment. A systems approach gives us the tools to explore the interconnections between farming and other aspects of our environment.
Everyone plays a role in creating a sustainable food system.
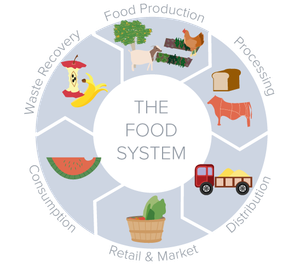
Making the transition to sustainable agriculture is a process. For farmers, the transition to sustainable agriculture normally requires a series of small , realistic steps . Family economics and personal goals influence how fast or how far participants can go in the transition. It is important to realize that each small decision can make a difference and contribute to advancing the entire system further on the "sustainable agriculture continuum." The key to moving forward is the will to take the next step. Finally, it is important to point out that reaching toward the goal of sustainable agriculture is the responsibility of all participants in the system , including farmers, laborers, policymakers, researchers, retailers, and consumers. Each group has its own part to play, its own unique contribution to make to strengthen the sustainable agriculture community. The remainder of this page considers specific strategies for realizing these broad themes or goals. The strategies are grouped according to three separate though related areas of concern: Farming and Natural Resources , Plant and Animal Production Practices , and the Economic, Social and Political Context . They represent a range of potential ideas for individuals committed to interpreting the vision of sustainable agriculture within their own circumstances.
- Farming and Natural Resources
When the production of food and fiber degrades the natural resource base, the ability of future generations to produce and flourish decreases. The decline of ancient civilizations in Mesopotamia, the Mediterranean region, Pre-Columbian southwest U.S. and Central America is believed to have been strongly influenced by natural resource degradation from non-sustainable farming and forestry practices.
Water is the principal resource that has helped agriculture and society to prosper, and it has been a major limiting factor when mismanaged.
Water supply and use. In California, an extensive water storage and transfer system has been established which has allowed crop production to expand to very arid regions. In drought years, limited surface water supplies have prompted overdraft of groundwater and consequent intrusion of salt water, or permanent collapse of aquifers. Periodic droughts, some lasting up to 50 years, have occurred in California.
Several steps should be taken to develop drought-resistant farming systems even in "normal" years, including both policy and management actions:
1) improving water conservation and storage measures,
2) providing incentives for selection of drought-tolerant crop species,
3) using reduced-volume irrigation systems,
4) managing crops to reduce water loss, or
5) not planting at all.
Water quality. The most important issues related to water quality involve salinization and contamination of ground and surface waters by pesticides, nitrates and selenium. Salinity has become a problem wherever water of even relatively low salt content is used on shallow soils in arid regions and/or where the water table is near the root zone of crops. Tile drainage can remove the water and salts, but the disposal of the salts and other contaminants may negatively affect the environment depending upon where they are deposited. Temporary solutions include the use of salt-tolerant crops, low-volume irrigation, and various management techniques to minimize the effects of salts on crops. In the long-term, some farmland may need to be removed from production or converted to other uses. Other uses include conversion of row crop land to production of drought-tolerant forages, the restoration of wildlife habitat or the use of agroforestry to minimize the impacts of salinity and high water tables. Pesticide and nitrate contamination of water can be reduced using many of the practices discussed later in the Plant Production Practices and Animal Production Practices sections.
Wildlife . Another way in which agriculture affects water resources is through the destruction of riparian habitats within watersheds. The conversion of wild habitat to agricultural land reduces fish and wildlife through erosion and sedimentation, the effects of pesticides, removal of riparian plants, and the diversion of water. The plant diversity in and around both riparian and agricultural areas should be maintained in order to support a diversity of wildlife. This diversity will enhance natural ecosystems and could aid in agricultural pest management.
Modern agriculture is heavily dependent on non-renewable energy sources, especially petroleum. The continued use of these energy sources cannot be sustained indefinitely, yet to abruptly abandon our reliance on them would be economically catastrophic. However, a sudden cutoff in energy supply would be equally disruptive. In sustainable agricultural systems, there is reduced reliance on non-renewable energy sources and a substitution of renewable sources or labor to the extent that is economically feasible.
Many agricultural activities affect air quality. These include smoke from agricultural burning; dust from tillage, traffic and harvest; pesticide drift from spraying; and nitrous oxide emissions from the use of nitrogen fertilizer. Options to improve air quality include:
- incorporating crop residue into the soil - using appropriate levels of tillage - and planting wind breaks, cover crops or strips of native perennial grasses to reduce dust.
Soil erosion continues to be a serious threat to our continued ability to produce adequate food. Numerous practices have been developed to keep soil in place, which include:
- reducing or eliminating tillage - managing irrigation to reduce runoff - and keeping the soil covered with plants or mulch.
Enhancement of soil quality is discussed in the next section.
- Plant Production Practices
Sustainable production practices involve a variety of approaches. Specific strategies must take into account topography, soil characteristics, climate, pests, local availability of inputs and the individual grower's goals. Despite the site-specific and individual nature of sustainable agriculture, several general principles can be applied to help growers select appropriate management practices:
- Selection of species and varieties that are well suited to the site and to conditions on the farm; - Diversification of crops (including livestock) and cultural practices to enhance the biological and economic stability of the farm; - Management of the soil to enhance and protect soil quality; - Efficient and humane use of inputs; and - Consideration of farmers' goals and lifestyle choices.
Selection of site, species and variety
Preventive strategies, adopted early, can reduce inputs and help establish a sustainable production system. When possible, pest-resistant crops should be selected which are tolerant of existing soil or site conditions. When site selection is an option, factors such as soil type and depth, previous crop history, and location (e.g. climate, topography) should be taken into account before planting.
Diversified farms are usually more economically and ecologically resilient. While monoculture farming has advantages in terms of efficiency and ease of management, the loss of the crop in any one year could put a farm out of business and/or seriously disrupt the stability of a community dependent on that crop. By growing a variety of crops, farmers spread economic risk and are less susceptible to the radical price fluctuations associated with changes in supply and demand.
Properly managed, diversity can also buffer a farm in a biological sense. For example, in annual cropping systems, crop rotation can be used to suppress weeds, pathogens and insect pests. Also, cover crops can have stabilizing effects on the agroecosystem by holding soil and nutrients in place, conserving soil moisture with mowed or standing dead mulches, and by increasing the water infiltration rate and soil water holding capacity. Cover crops in orchards and vineyards can buffer the system against pest infestations by increasing beneficial arthropod populations and can therefore reduce the need for chemical inputs. Using a variety of cover crops is also important in order to protect against the failure of a particular species to grow and to attract and sustain a wide range of beneficial arthropods.
Optimum diversity may be obtained by integrating both crops and livestock in the same farming operation. This was the common practice for centuries until the mid-1900s when technology, government policy and economics compelled farms to become more specialized. Mixed crop and livestock operations have several advantages. First, growing row crops only on more level land and pasture or forages on steeper slopes will reduce soil erosion. Second, pasture and forage crops in rotation enhance soil quality and reduce erosion; livestock manure, in turn, contributes to soil fertility. Third, livestock can buffer the negative impacts of low rainfall periods by consuming crop residue that in "plant only" systems would have been considered crop failures. Finally, feeding and marketing are flexible in animal production systems. This can help cushion farmers against trade and price fluctuations and, in conjunction with cropping operations, make more efficient use of farm labor.
Soil management
A common philosophy among sustainable agriculture practitioners is that a "healthy" soil is a key component of sustainability; that is, a healthy soil will produce healthy crop plants that have optimum vigor and are less susceptible to pests. While many crops have key pests that attack even the healthiest of plants, proper soil, water and nutrient management can help prevent some pest problems brought on by crop stress or nutrient imbalance. Furthermore, crop management systems that impair soil quality often result in greater inputs of water, nutrients, pesticides, and/or energy for tillage to maintain yields.
In sustainable systems, the soil is viewed as a fragile and living medium that must be protected and nurtured to ensure its long-term productivity and stability. Methods to protect and enhance the productivity of the soil include:
- using cover crops, compost and/or manures - reducing tillage - avoiding traffic on wet soils - maintaining soil cover with plants and/or mulches
Conditions in most California soils (warm, irrigated, and tilled) do not favor the buildup of organic matter. Regular additions of organic matter or the use of cover crops can increase soil aggregate stability, soil tilth, and diversity of soil microbial life.
Efficient use of inputs
Many inputs and practices used by conventional farmers are also used in sustainable agriculture. Sustainable farmers, however, maximize reliance on natural, renewable, and on-farm inputs. Equally important are the environmental, social, and economic impacts of a particular strategy. Converting to sustainable practices does not mean simple input substitution. Frequently, it substitutes enhanced management and scientific knowledge for conventional inputs, especially chemical inputs that harm the environment on farms and in rural communities. The goal is to develop efficient, biological systems which do not need high levels of material inputs.
Growers frequently ask if synthetic chemicals are appropriate in a sustainable farming system. Sustainable approaches are those that are the least toxic and least energy intensive, and yet maintain productivity and profitability. Preventive strategies and other alternatives should be employed before using chemical inputs from any source. However, there may be situations where the use of synthetic chemicals would be more "sustainable" than a strictly non-chemical approach or an approach using toxic "organic" chemicals. For example, one grape grower switched from tillage to a few applications of a broad spectrum contact herbicide in the vine row. This approach may use less energy and may compact the soil less than numerous passes with a cultivator or mower.
Consideration of farmer goals and lifestyle choices
Management decisions should reflect not only environmental and broad social considerations, but also individual goals and lifestyle choices. For example, adoption of some technologies or practices that promise profitability may also require such intensive management that one's lifestyle actually deteriorates. Management decisions that promote sustainability, nourish the environment, the community and the individual.
- Animal Production Practices
In the early part of this century, most farms integrated both crop and livestock operations. Indeed, the two were highly complementary both biologically and economically. The current picture has changed quite drastically since then. Crop and animal producers now are still dependent on one another to some degree, but the integration now most commonly takes place at a higher level-- between farmers, through intermediaries, rather than within the farm itself. This is the result of a trend toward separation and specialization of crop and animal production systems. Despite this trend, there are still many farmers, particularly in the Midwest and Northeastern U.S. that integrate crop and animal systems--either on dairy farms, or with range cattle, sheep or hog operations.
Even with the growing specialization of livestock and crop producers, many of the principles outlined in the crop production section apply to both groups. The actual management practices will, of course, be quite different. Some of the specific points that livestock producers need to address are listed below.
Management Planning
Including livestock in the farming system increases the complexity of biological and economic relationships. The mobility of the stock, daily feeding, health concerns, breeding operations, seasonal feed and forage sources, and complex marketing are sources of this complexity. Therefore, a successful ranch plan should include enterprise calendars of operations, stock flows, forage flows, labor needs, herd production records and land use plans to give the manager control and a means of monitoring progress toward goals.
Animal Selection
The animal enterprise must be appropriate for the farm or ranch resources. Farm capabilities and constraints such as feed and forage sources, landscape, climate and skill of the manager must be considered in selecting which animals to produce. For example, ruminant animals can be raised on a variety of feed sources including range and pasture, cultivated forage, cover crops, shrubs, weeds, and crop residues. There is a wide range of breeds available in each of the major ruminant species, i.e., cattle, sheep and goats. Hardier breeds that, in general, have lower growth and milk production potential, are better adapted to less favorable environments with sparse or highly seasonal forage growth.
Animal nutrition
Feed costs are the largest single variable cost in any livestock operation. While most of the feed may come from other enterprises on the ranch, some purchased feed is usually imported from off the farm. Feed costs can be kept to a minimum by monitoring animal condition and performance and understanding seasonal variations in feed and forage quality on the farm. Determining the optimal use of farm-generated by-products is an important challenge of diversified farming.
Reproduction
Use of quality germplasm to improve herd performance is another key to sustainability. In combination with good genetic stock, adapting the reproduction season to fit the climate and sources of feed and forage reduce health problems and feed costs.
Herd Health
Animal health greatly influences reproductive success and weight gains, two key aspects of successful livestock production. Unhealthy stock waste feed and require additional labor. A herd health program is critical to sustainable livestock production.
Grazing Management
Most adverse environmental impacts associated with grazing can be prevented or mitigated with proper grazing management. First, the number of stock per unit area (stocking rate) must be correct for the landscape and the forage sources. There will need to be compromises between the convenience of tilling large, unfenced fields and the fencing needs of livestock operations. Use of modern, temporary fencing may provide one practical solution to this dilemma. Second, the long term carrying capacity and the stocking rate must take into account short and long-term droughts. Especially in Mediterranean climates such as in California, properly managed grazing significantly reduces fire hazards by reducing fuel build-up in grasslands and brushlands. Finally, the manager must achieve sufficient control to reduce overuse in some areas while other areas go unused. Prolonged concentration of stock that results in permanent loss of vegetative cover on uplands or in riparian zones should be avoided. However, small scale loss of vegetative cover around water or feed troughs may be tolerated if surrounding vegetative cover is adequate.
Confined Livestock Production
Animal health and waste management are key issues in confined livestock operations. The moral and ethical debate taking place today regarding animal welfare is particularly intense for confined livestock production systems. The issues raised in this debate need to be addressed.
Confinement livestock production is increasingly a source of surface and ground water pollutants, particularly where there are large numbers of animals per unit area. Expensive waste management facilities are now a necessary cost of confined production systems. Waste is a problem of almost all operations and must be managed with respect to both the environment and the quality of life in nearby communities. Livestock production systems that disperse stock in pastures so the wastes are not concentrated and do not overwhelm natural nutrient cycling processes have become a subject of renewed interest.
- The Economic, Social & Political Context
In addition to strategies for preserving natural resources and changing production practices, sustainable agriculture requires a commitment to changing public policies, economic institutions, and social values. Strategies for change must take into account the complex, reciprocal and ever-changing relationship between agricultural production and the broader society.
The "food system" extends far beyond the farm and involves the interaction of individuals and institutions with contrasting and often competing goals including farmers, researchers, input suppliers, farmworkers, unions, farm advisors, processors, retailers, consumers, and policymakers. Relationships among these actors shift over time as new technologies spawn economic, social and political changes.
A wide diversity of strategies and approaches are necessary to create a more sustainable food system. These will range from specific and concentrated efforts to alter specific policies or practices, to the longer-term tasks of reforming key institutions, rethinking economic priorities, and challenging widely-held social values. Areas of concern where change is most needed include the following:
Food and agricultural policy
Existing federal, state and local government policies often impede the goals of sustainable agriculture. New policies are needed to simultaneously promote environmental health, economic profitability, and social and economic equity. For example, commodity and price support programs could be restructured to allow farmers to realize the full benefits of the productivity gains made possible through alternative practices. Tax and credit policies could be modified to encourage a diverse and decentralized system of family farms rather than corporate concentration and absentee ownership. Government and land grant university research policies could be modified to emphasize the development of sustainable alternatives. Marketing orders and cosmetic standards could be amended to encourage reduced pesticide use. Coalitions must be created to address these policy concerns at the local, regional, and national level.
Conversion of agricultural land to urban uses is a particular concern in California, as rapid growth and escalating land values threaten farming on prime soils. Existing farmland conversion patterns often discourage farmers from adopting sustainable practices and a long-term perspective on the value of land. At the same time, the close proximity of newly developed residential areas to farms is increasing the public demand for environmentally safe farming practices. Comprehensive new policies to protect prime soils and regulate development are needed, particularly in California's Central Valley. By helping farmers to adopt practices that reduce chemical use and conserve scarce resources, sustainable agriculture research and education can play a key role in building public support for agricultural land preservation. Educating land use planners and decision-makers about sustainable agriculture is an important priority.
In California, the conditions of agricultural labor are generally far below accepted social standards and legal protections in other forms of employment. Policies and programs are needed to address this problem, working toward socially just and safe employment that provides adequate wages, working conditions, health benefits, and chances for economic stability. The needs of migrant labor for year-around employment and adequate housing are a particularly crucial problem needing immediate attention. To be more sustainable over the long-term, labor must be acknowledged and supported by government policies, recognized as important constituents of land grant universities, and carefully considered when assessing the impacts of new technologies and practices.
Rural Community Development
Rural communities in California are currently characterized by economic and environmental deterioration. Many are among the poorest locations in the nation. The reasons for the decline are complex, but changes in farm structure have played a significant role. Sustainable agriculture presents an opportunity to rethink the importance of family farms and rural communities. Economic development policies are needed that encourage more diversified agricultural production on family farms as a foundation for healthy economies in rural communities. In combination with other strategies, sustainable agriculture practices and policies can help foster community institutions that meet employment, educational, health, cultural and spiritual needs.
Consumers and the Food System
Consumers can play a critical role in creating a sustainable food system. Through their purchases, they send strong messages to producers, retailers and others in the system about what they think is important. Food cost and nutritional quality have always influenced consumer choices. The challenge now is to find strategies that broaden consumer perspectives, so that environmental quality, resource use, and social equity issues are also considered in shopping decisions. At the same time, new policies and institutions must be created to enable producers using sustainable practices to market their goods to a wider public. Coalitions organized around improving the food system are one specific method of creating a dialogue among consumers, retailers, producers and others. These coalitions or other public forums can be important vehicles for clarifying issues, suggesting new policies, increasing mutual trust, and encouraging a long-term view of food production, distribution and consumption.
Contributors : Written by Gail Feenstra , Writer; Chuck Ingels, Perennial Cropping Systems Analyst; and David Campbell, Economic and Public Policy Analyst with contributions from David Chaney, Melvin R. George, Eric Bradford, the staff and advisory committees of the UC Sustainable Agriculture Research and Education Program.
How to cite this page UC Sustainable Agriculture Research and Education Program. 2021. "What is Sustainable Agriculture?" UC Agriculture and Natural Resources. <https://sarep.ucdavis.edu/sustainable-ag>
This page was last updated August 3, 2021.
What is Sustainable Agriculture and Why Is It Important?
A new approach to agriculture is taking hold across the United States—an approach that has the potential to transform our entire food system for the better.
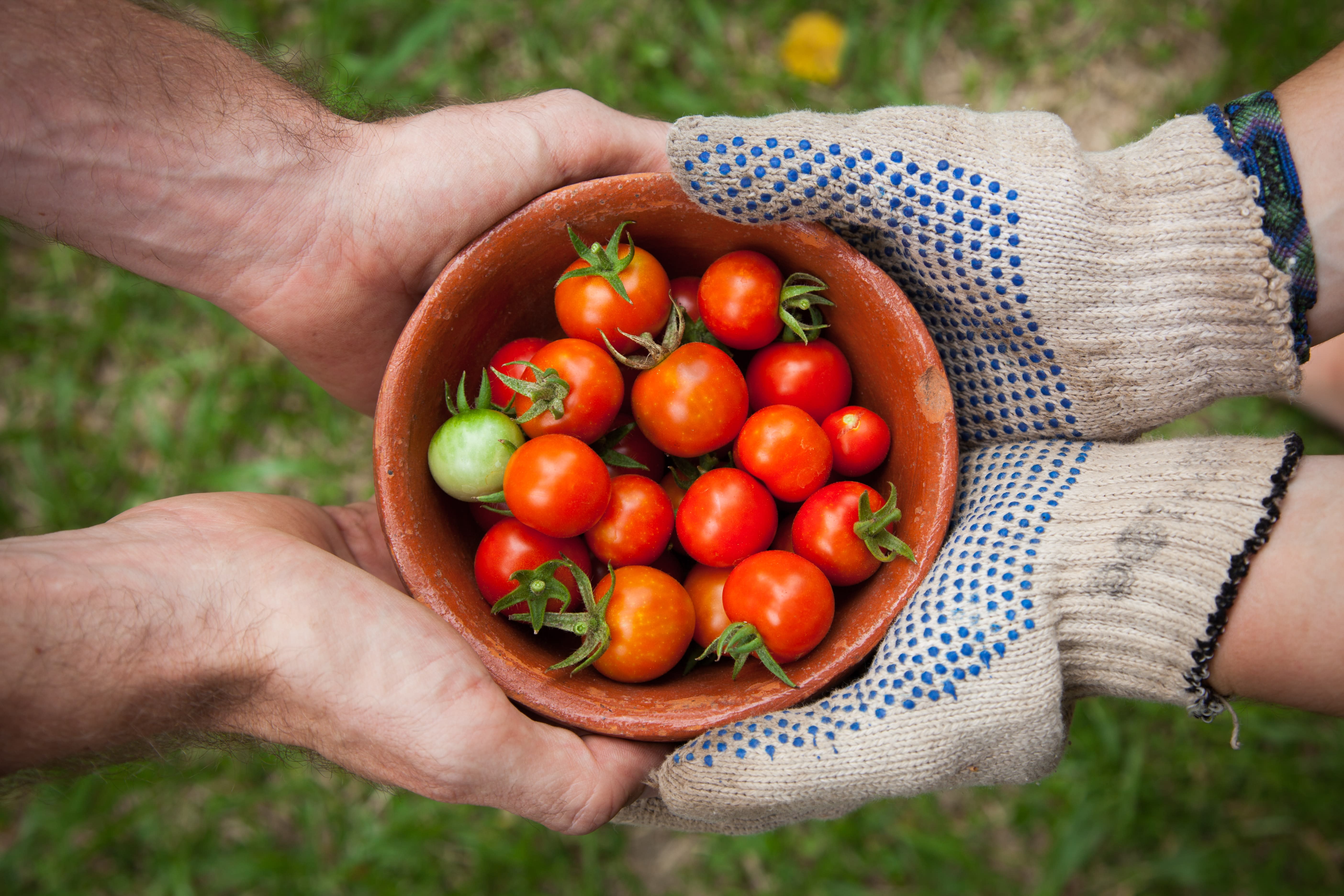
A few years ago, if you had opened the doors to a chicken house on Halley Farm in Texas, you would have been hit with the stench of ammonia. You would have heard—and then, as your eyes adjusted to the darkness, you would have seen—thousands of chickens packed into a vast warehouse. Looking closer, you would have noticed countless animals lying on the ground, nursing broken bones and painfully raw patches of skin, all of them suffering. You would have seen a system that was, to put it simply, unsustainable.
Today, if you open those same doors, you’ll see rows and rows of green hemp plants drying after harvest, providing a long-term source of revenue for the farm. You’ll see a system better for the Halley family, better for the community, better for the environment, and—of course—better for the animals. Halley Farm is a member of Mercy for Animals’ Transfarmation Project , just one example in the widespread movement toward a more sustainable agricultural system.
What is sustainable agriculture?
“Sustainable agriculture” simply refers to farming practices that incorporate and prioritize sustainability, or the ability to maintain a process over time. It describes an agricultural system that can be sustained indefinitely, providing food and fiber to meet the needs of the present and the needs of future generations. Author and environmentalist Wes Jackson is credited with having coined the term in his 1980 book New Roots for Agriculture—and the buzzy phrase has since been used (sometimes inaccurately) in books, in films and documentaries, on product packaging, in news articles, at industry summits, and even in US law.
Practitioners of sustainable agriculture take a holistic viewpoint. Rather than just aiming to maximize profit and production in the short term, they prioritize the longevity of the system—considering how their farming practices impact the environment, local communities, animals, and every person they encounter on the journey from farm to table. Rather than working against nature—destroying forested landscapes , killing insects with harmful pesticides , and depleting soil with chemical fertilizer—sustainable agriculture aims to work with the natural landscape, creating a system that’s built to last.
Although the word “sustainable” often has environmental connotations, it can also refer to societies, economies, and ways of life. That’s why the main idea behind sustainable agriculture is to steward both natural resources and human resources—protecting the wellbeing of farmers, strengthening local economies, improving living conditions in surrounding communities, promoting consumer health, and much more.
While proponents of animal farming often characterize some forms of animal agriculture as being “sustainable” (such as the popular idea of regenerative ranching ), it’s important to note that livestock-based systems are inherently unsustainable. Not only does cattle ranching waste valuable land and water by growing crops for animal feed rather than human consumption, but the concept of using cattle to support carbon sequestration also faces significant issues of scale. Dr. Tara Garnett of the University of Oxford, who led an exhaustive research report on regenerative ranching, points out that "rising animal production and consumption, whatever the farming system and animal type , is causing damaging greenhouse gas release and contributing to changes in land use.” A truly sustainable system of agriculture uses as few resources as possible, while causing the least amount of harm and destruction—which ultimately means a system focused on growing plants.
Why is sustainable agriculture important?
Starting in the early 1900s, as the Industrial Revolution blossomed across the United States, farms began to look different. Flourishing, colorful fields bursting with a variety of fruits and vegetables came to be replaced by monocultures —vast fields of a single, identical crop. Nutrient-rich soil, once nourished by garden compost, became thin and lifeless. And picturesque farm fields were replaced by enormous windowless sheds , or Concentrated Animal Feeding Operations (CAFOs)—all packed as tightly as possible with suffering animals.
Industrial agriculture had become the new face of the US food system, characterized by large-scale monocultures used for animal feed, high levels of chemical pesticides and fertilizers, and CAFOs where living beings were treated as nothing more than products. Instead of being run by small farmers, these machine-like operations were managed by giant corporations wielding inordinate amounts of money and political power. This was the case for Halley Farm, which was locked into a contract with Pilgrim’s Pride —leaving the family with sick chickens, their own injuries and health complications from working with the animals in hazardous conditions, and hundreds of thousands of dollars of debt.
The system of intensive agriculture described above aims to maximize profits and production—all with blatant disregard for the climate, soils, water, humans, and animals involved. Along with relying on systemic cruelty toward people and animals, industrial animal agriculture has an enormous carbon footprint , with some estimates showing food systems are responsible for one-third of greenhouse gas emissions. So while this current system meets our immediate needs, it’s destroying the very environment and resources it relies on.
Sustainable agriculture represents a paradigm shift: Rather than extracting as many resources as possible, it aims to work in harmony with the natural environment, creating a self-sustaining system that will endure for generations to come. This means shifting away from our current system of intensive, animal-focused agriculture and toward a more efficient, harmonious, plant-focused system. Notably, sustainable and plant-centric agricultural practices can help mitigate catastrophic climate change , while also remaining resilient in the face of extreme weather, drought, and flooding.
Here are just a few of the benefits of sustainable agriculture.
1. Saving water
Intensive animal agriculture wastes enormous amounts of water. Every day, a single dairy cow uses between 40 and 50 gallons . On top of that, growing the crops used for animal feed is incredibly water-intensive—so much so that animal agriculture is responsible for nearly 20% of freshwater use globally. By contrast, sustainable farming practices view water as the precious resource it is, aiming to conserve whenever possible—through smarter irrigation systems, drought-tolerant plants, cover crops to support soil structure, and moving away from animal farming altogether.
2. Reducing pollution
Along with wasting water, factory farming pollutes critical water sources—with agricultural runoff listed among the leading causes of water pollution in the US. High concentrations of animals on these farms result in enormous volumes of waste, all rich in phosphorus and nitrogen. When this pollution seeps into the groundwater and nearby bodies of water, it causes enormous “ dead zones ,” or algae blooms that kill fish and other marine life.
Factory farming also pollutes the very air we breathe —whether from the toxic ammonia emanating from poultry farms, massive amounts of pesticides sprayed on crops grown for animal feed, or emissions of nitrous oxide from nitrogen fertilizer. Air pollution harms the health of the animals, farm workers, and even the surrounding community. By transitioning to more sustainable and plant-centric agricultural practices, farmers can help ensure clean air and water for future generations.
3. Increasing resilience to extreme weather
“Agriculture is a risky business,” Laura Lengnick points out in her Introduction to Climate Resilience in Agriculture, a resource for farmers. “The outcome of every growing season hinges on dynamic interactions between soils, crops, livestock, pests, weather, finances, regulations and markets… But in recent years, managing weather-related risk, already one of the highest risk factors in agriculture, has become even harder , as weather patterns become more variable from day to day and season to season.”
As the impacts of climate change continue to worsen, the farms that grow our food need to be able to withstand drought, wildfires, flooding, and other forms of extreme weather. Through sustainable practices, many farmers are working to increase the resilience of their systems. Methods include diversifying crops (which reduces economic vulnerability), adding improved drainage or irrigation systems, protecting and strengthening soil by planting cover crops, and more.
4. Protecting soil health and storing carbon
Soil itself is a living ecosystem that supports plants, animals, and humans. It’s the foundation of our life on earth! And, as is the case for any living being, the overall health of soil is of utmost importance. Healthy soil should be able to effectively cycle nutrients to make them available to plants, maintain a strong enough structure to hold water, filter contaminants to clean the freshwater supply, and withstand erosion. Protecting soil health is a key component of sustainable agriculture, which seeks to ensure the longevity of soil rather than depleting it for immediate gain.
Perhaps the most magical thing about healthy soil is that it works as a carbon sink, sequestering carbon from the atmosphere. So not only does protecting soil increase its resilience to extreme weather, it also helps mitigate the worst effects of climate change.
What is the main goal of sustainable agriculture?
The main goal of sustainable agriculture is simply to ensure that our food system will continue to effectively provide for future generations—even within the evolving conditions caused by global climate change. Rather than solely focusing on profits, sustainable agriculture practitioners aim to steward the environment, advance social equity, and strengthen local economies—all while profiting economically to sustain their work for the long term.
As noted in the USDA’s National Agricultural Library: “If advocating the need for a sustainable agriculture has become universal, agreement as to what is required to achieve it has not .” Despite the rich conversation it has inspired over the last few decades, sustainable agriculture still lacks a single definition or clear standard. While a few groups have attempted to define a set of guidelines and provide “sustainable agriculture” certifications, including the Rainforest Alliance , the term’s official meaning is still under debate. US Congress first addressed the concept of sustainable agriculture by attempting to define it in the 1990 “Farm Bill”—but their definition, according to US Code Title 7, Section 3103, still suggests that animal production practices can be sustainable:
“An integrated system of plant and animal production practices having a site-specific application that will, over the long-term— (A) satisfy human food and fiber needs (B) enhance environmental quality and the natural resource base upon which the agriculture economy depends (C) make the most efficient use of nonrenewable resources and on-farm resources and integrate, where appropriate, natural biological cycles and controls (D) sustain the economic viability of farm operations (E) enhance the quality of life for farmers and society as a whole.”
There’s still room in the discussion for a plant-centric definition of sustainable agriculture, one that prioritizes productivity, efficiency, and longevity—and not at the expense of animals, people, or the environment.
What are sustainable agriculture practices?
However it’s defined, sustainable agriculture should be thought of as an ongoing practice and philosophy, guiding all decisions and processes on a farm. Here are some of the ways farmers can practice sustainable agriculture on a day-to-day basis.
"No-till" farming
Remember the importance of protecting soil health? By reducing or eliminating tillage (the traditional method of plowing a garden), farmers avoid disturbing the soil, helping to reduce erosion and increase biological activity within the soil. According to the USDA, soil left to its own biological processes often has a higher organic matter content than conventionally tilled soil. This method also saves money and time, which is more sustainable for farmers as well.
Planting cover crops and perennials
Another way to protect, nourish, and restore soil is to plant cover crops . Generally, these crops are planted in the fall or winter to protect vulnerable soil from wind and snow. By creating a protective canopy, cover crops reduce soil runoff and lock in nutrients—resulting in better soil structure, stability, and moisture over time. Common cover crops include clovers, turnips, mustard, buckwheat, alfalfa, and radishes.
Integrated pest management (IPM)
Integrated pest management (IPM) is a fancy way of saying… protecting gardens from bugs! Rather than spraying harmful pesticides, IPM takes the holistic viewpoint that is characteristic of sustainable agriculture. It encourages creative solutions like disrupting the pest’s environment, bringing in “natural enemies” (which might even be other insects!), planting crops that discourage certain bugs, and closely monitoring the situation to accurately diagnose and predict pest problems. While IPM sounds more labor-intensive than spraying pesticides, it actually aims to find the most effective and least-risk option, preventing further problems (and more work) later on. Along with its agricultural applications, IPM can also be used to manage pests in homes, businesses, and recreational areas.
Rotating crops and encouraging diversity
Ecosystems are meant to exist as interdependent, resilient communities, with diverse forms of life supporting each other’s various processes. But intensive agriculture today looks very different. Always profit- and efficiency-driven, it prioritizes monocultures, or vast fields of a single crop—usually corn, soybeans, or wheat. These plants are especially vulnerable to disease and pests, as they aren’t supported by the biodiversity that protects plants in the natural world.
Farmers can change this through polyculture and crop rotation, which supports the soil and helps suppress disease and pest infestations. Diversified farms are also more economically resilient, protecting farmers from becoming totally dependent on a single vulnerable crop—and from price fluctuations associated with evolving supply and demand.
Adopting agroforestry practices
Agroforestry is when a farmer intentionally integrates trees into their crop systems. This could mean planting crops in an area protected by trees, known as a “windbreak;” growing crops under a forest canopy for shade; or planting trees and shrubs along a river to prevent erosion and filter farm runoff. With their sturdy roots and the protection from wind, sun, and rain they provide, trees offer a wonderful way to work in harmony with the natural landscape.
What is the current greatest threat to agricultural sustainability?
Unfortunately, the existing system of intensive animal agriculture is incredibly powerful—and so is its marketing. In the last few years, phrases like “grass-fed beef,” “regeneratively grown,” and “sustainably sourced” have popped up all over store shelves. The idea that regenerative ranching could provide a climate solution has given the meat industry a convenient excuse to keep producing hamburgers—and emissions. Switching to grass-fed beef is simply not a solution . Instead, opting for a plant-based diet can keep money out of the pockets of Big Ag, instead diverting it to projects like Halley Farm that are working to diversify and strengthen our food system.
A food system for the future
Picture a lush and vibrant landscape bursting with a variety of trees, vegetables, and native plants. Beneath the surface, the rich soil is busy cycling nutrients and sequestering carbon from the atmosphere. Above ground, the lush plants exhale oxygen and provide nourishing food for insects, animals, and humans. Trees offer generous shade, wild birds and animals take shelter in the plant life, and bright flowers create a peaceful oasis for all to enjoy.
This is the future that sustainable agriculture imagines—and the future that the Halley Farm family, in partnership with Mercy for Animals, is trying to work toward. By transitioning from raising poultry to growing hemp, they’ve experienced not only better financial investment and payoff, but also a much higher quality of life on the farm.
“It’s been really great to see Bo and Sam go from very troubled, angry, and broken people while the chicken houses were here to just flourishing with joy working with the plants,” says family friend Evan about the two brothers. “It just melts your heart... How can you not love that?”
What you can do
As a consumer, you have tremendous power. Simply cutting back on animal products can help divert money away from industrial agriculture—revoking support for factory farming and the monocultures used to grow animal feed. Instead, opt for a diverse, colorful diet of fruits, vegetables, grains, and legumes. Diversifying your plate is a simple way to support a more diversified, sustainable food system.
The large-scale transition to a more sustainable food system won’t happen in a day. But in the meantime, we can end the worst forms of abuse—especially for animals like chickens, who account for 9 in 10 land animals raised for food around the world. Join us in demanding that corporate giants sign onto the Better Chicken Commitment and end the most extreme forms of animal abuse.
More like this
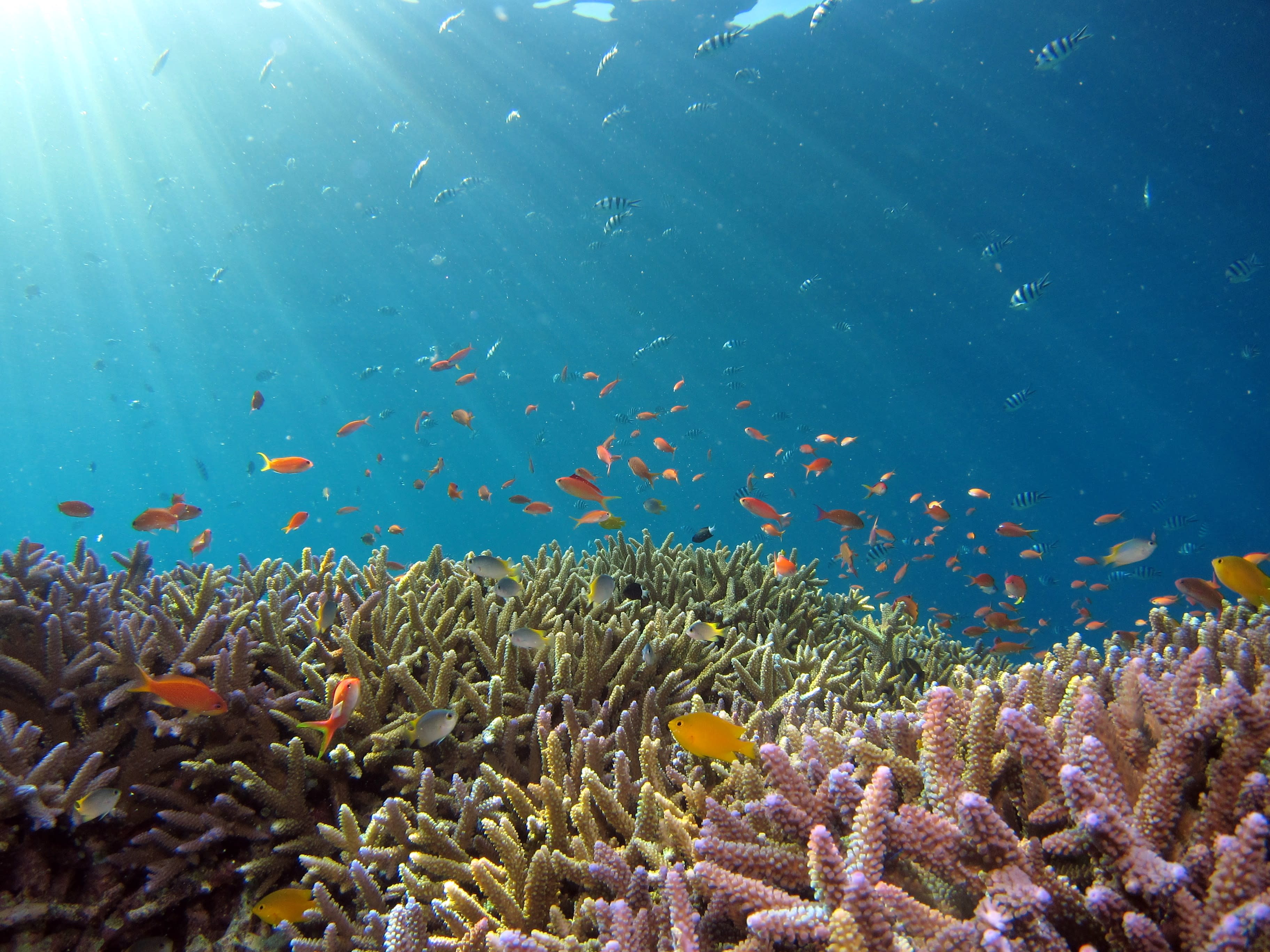
What is ocean conservation and why is it more important than ever?
To protect the planet, humans must begin at the source: the ocean.
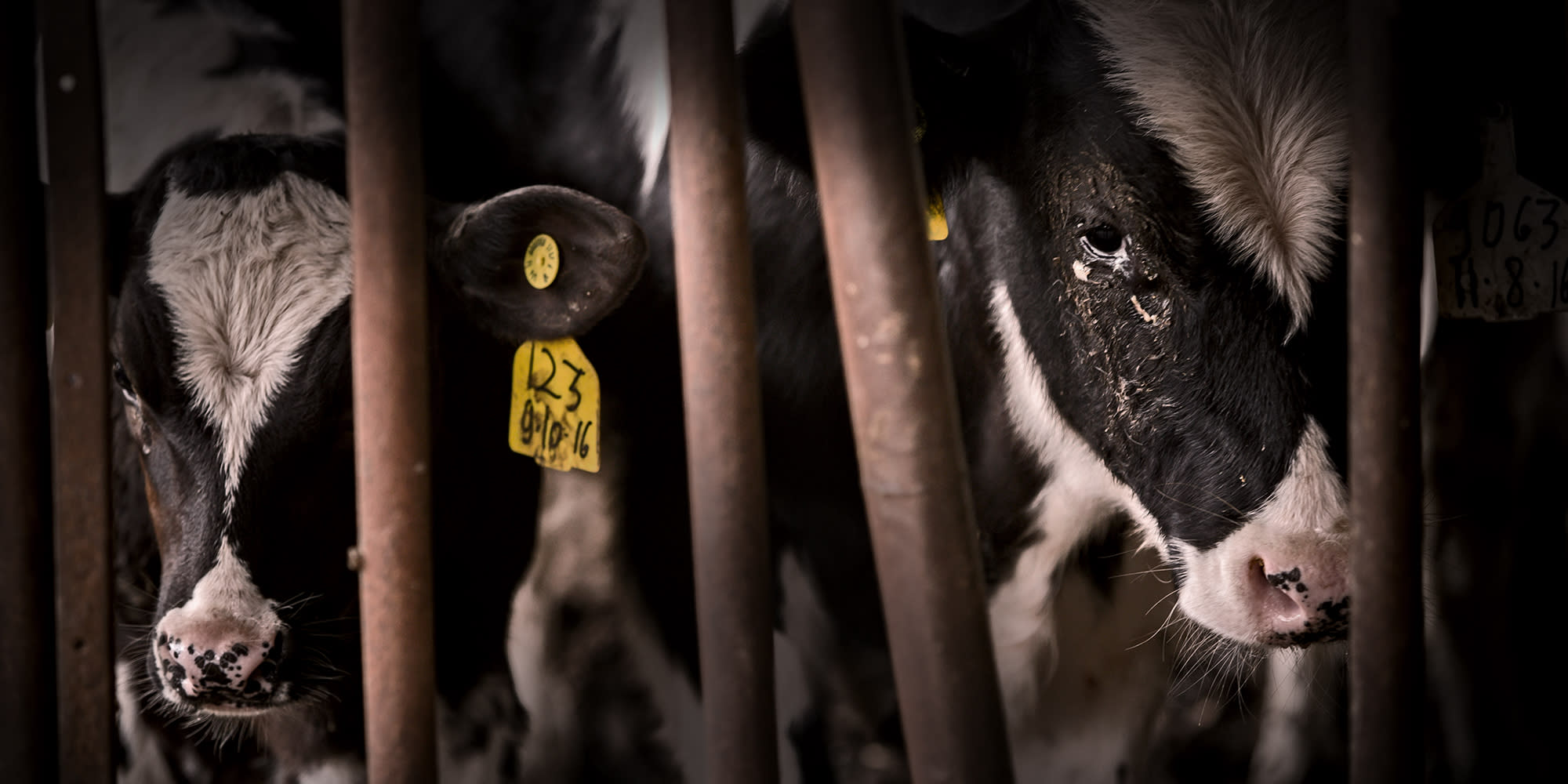
Industrial Agriculture is Far Worse than You Think
Mutilated animals. Dead zones. Sewage lagoons. Pandemic factories. It sounds like something out of a dystopian novel, but it's happening right now—all thanks to an industry that values profit over life on Earth.
Essay on Sustainable Agriculture
Introduction: what is sustainable agriculture, importance of sustainable agriculture, population growth, per capita food consumption, sustainable agriculture and technology, green politics, conclusion of sustainable agriculture.
Bibliography
Sustainable agriculture has dominated the sociological understanding of the rural world largely. Following the enthusiasm around the concept as a means of eradication of poverty and turning the economy to a “resource-efficient, low carbon Green Economy” 1 . Global population, and consequently consumption has increased.
However, technology development has matched the demand for food in terms of food production, but the distribution of food is not evenly distributed. This has brought forth the question of the possibility of supplying adequate food to the ever-growing global population.
Further, the challenges posed by depleting non-renewable sources of energy, rising costs, and climate change has brought the issue related to sustainability of food production and the related social and economic impact of the food production into forefront. This paper outlines the meaning and technology related to sustainable agriculture and tries to gauge its impact as a possible solution to the impending food crisis.
Sustainable agriculture is a process of farming using eco-friendly methods understanding and maintaining the relationship between the organisms and environment. In this process of agriculture and animal husbandry are combined to form a simultaneous process and practice. In other words, sustainable agriculture is an amalgamation of three main elements viz. ecological health, profitability, and propagating equality.
The concept of sustainability rests on the principle of not wasting any resources that may become useful to the future generation. Therefore, the main idea of sustainability rests on stewardship of individual and natural resources. Before understanding the technology involved in sustainable agriculture, it is important to know why we need it in the first place.
The rise in population growth and urbanization of people has led to a dietary change of the world population, which now rests more on animal protein 2 . Therefore understanding the demographic changes in the world population has become an important parameter to judge the future demand for food.
As population growth rate is the key variable that affects the demand for food, therefore understanding the number of people increasing worldwide is important. According to the UNDP results, the annual population growth rate had declined from 2.2% in 1962 to 1.1% in 2010, however, this increase to indicate an increase of 75 million people 3 .
However, this increase in population is not equitably distributed as some areas such as Africa, Latin America, and Asia face a growth rate of 2% while others such as the erstwhile Soviet bloc countries have a negative rate.
According to the UNDP predictions, population worldwide is expected to increase to 9 billion in 2050 from the present 7 billion 4 . Therefore, the uncertain growth in population is expected to affect food demand and therefore food production.
Undernourishment is a prevalent problem in the developing world, wherein almost 20% of the developing world that is more than 5 billion people is undernourished.
Further, in emerging economies, food consumption is increasing with increased preference for animal protein such as meat, dairy products, and egg. Therefore, the growth of consumption of animal protein has increased the necessity of grazing of livestock, therefore, increasing further pressure on the food supply.
It is believed that the increase in the demand for food due to the increase in global population and change in dietary habit of the population. In the past, the demand for food and the rate of production has remained at par, but the unequal distribution of food has led to the major problem in food supply and starvation in various parts of the world.
Another problem that food production in the future faces is the constraint of non-renewable natural resources. The most critical resources, which are becoming scant for the future generations are –
- Land : Availability of land globally to cultivate food has grown marginally due to the increase in global population. The availability of land available per person to grow food has declined from 1.30 hectares in 1967 to 0.72 hectares in 2007 5 . Therefore, a clear dearth in agricultural land is a deterrent to future agriculture.
- Water : The world comprises of 70% freshwater resources, available from river and groundwater. Deficiency of freshwater has been growing as usage of water has increased more than twice the rate of population growth 6 . As water is required for irrigation purposes, water availability to is not equally distributed around the world. Therefore, reduced water supply would limit the per capita production of food.
- Energy : Globally, the scarcity of the non-renewable resources of energy is another concern. The global demand for energy is expected to double by 2050, consequently increasing energy prices 7 . Therefore, food production for the future will have to devise a technology based on renewable sources of energy.
The question of sustainability in agriculture arose due to some pressing issues that have limited the utilization of erstwhile processes and technologies for food production. However, it should be noted that sustainable agriculture does not prescribe any set rule or technology for the production process, rather shows a way towards sustainability 8 .
Sustainable agriculture uses best management practice by adhering to target-oriented cultivation. The agriculture process looks at disease-oriented hybrid, pest control through use of biological insecticides and low usage of chemical pesticide and fertilizer. Usually, insect-specific pest control is used, which is biological in nature.
Water given to the crops is through micro-sprinklers which help is directly watering the roots of the plants, and not flooding the field completely. The idea is to manage the agricultural land for both plants and animal husbandry.
For instance, in many southwestern parts of Florida’s citrus orchards, areas meant for water retention and forest areas become a natural habitat for birds and other animals 9 . The process uses integrated pest management that helps in reducing the amount of pesticide used in cultivation.
Sustainable agriculture adopts green technology as a means of reducing wastage of non-renewable energy and increase production. In this respect, the sustainable agricultural technology is linked to the overall developmental objective of the nation and is directly related to solving socio-economic problems of the nation 10 .
The UN report states, “The productivity increases in possible through environment-friendly and profitable technologies.” 11 In order to understand the technology better, one must realize that the soil’s health is crucial for cultivation of crops.
Soil is not just another ingredient for cultivation like pesticides or fertilizers; rather, it is a complex and fragile medium that must be nurtured to ensure higher productivity 12 . Therefore, the health of the soil can be maintained using eco-friendly methods:
Healthy soil, essential to agriculture, is a complex, living medium. The loose but coherent structure of good soil holds moisture and invites airflow. Ants (a) and earthworms (b) mix the soil naturally. Rhizobium bacteria (c) living in the root nodules of legumes (such as soybeans) create fixed nitrogen, an essential plant nutrient.
Other soil microorganisms, including fungi (d), actinomycetes (e) and bacteria (f), decompose organic matter, thereby releasing more nutrients. Microorganisms also produce substances that help soil particles adhere to one another. To remain healthy, soil must be fed organic materials such as various manures and crop residues. 13
This is nothing but a broader term to denote environment-friendly solutions to agricultural production. Therefore, the technology-related issue of sustainable agriculture is that it should use such technology that allows usage of renewable sources of energy and is not deterrent to the overall environment.
The politics around sustainable agriculture lies in the usage of the renewable sources of energy and disciplining of the current consumption rates 14 . The politics related to the sustainable agriculture is also related to the politics of sustainable consumption.
Though there is a growing concern over depleting food for the future and other resources, there is hardly any measure imposed by the governments of developed and emerging economies to sustain the consumption pattern of the population 15 .
The advocates of green politics believe that a radical change of the conventional agricultural process is required for bringing forth sustainable agriculture 16 . Green politics lobbies for an integrated farming system that can be the only way to usher in sustainable agricultural program 17 .
Sustainable agriculture is the way to maintain a parity between the increasing pressure of food demand and food production in the future. As population growth, change in income demographics, and food preference changes, there are changes in the demand of food of the future population.
Further, changes in climate and increasing concern regarding the depletion of non-renewable sources of energy has forced policymakers and scientists to device another way to sustain the available resources as well as continue meeting the increased demand of food.
Sustainable agriculture is the method through which these problems can be overlooked, bringing forth a new integrated form of agriculture that looks at food production in a holistic way.
Batie, S. S., ‘Sustainable Development: Challenges to Profession of Agricultural Economics’, American Journal of Agricultural Economics, vol. 71, no. 5, 1989: 1083-1101.
Dobson, A., The Politics of Nature: Explorations in Green Political Theory, Psychology Press, London, 1993.
Leaver, J. D., ‘Global food supply: a challenge for sustainable agriculture’, Nutrition Bulletin, vol. 36 , 2011: 416-421.
Martens, S., & G. Spaargaren, ‘The politics of sustainable consumption: the case of the Netherlands’, Sustainability: Science, Practice, & Policy, vol.1 no. 1, 2005: 29-42.
Morris, C., & M. Winter, ‘Integrated farming systems: the third way for European agriculture?’, Land Use Policy, vol. 16, no. 4, 1999: 193–205.
Reganold, J. P., R. I. Papendick, & J. F. Parr, ‘Sustainable Agriculture’, Scientific American , 1990: 112-120.
Townsend, C., ‘ Technology for Sustainable Agriculture. ‘ Florida Gulf Coast University, 1998. Web.
United Nations, ‘ Green technology for sustainable agriculture development ‘, United Nations Asian And Pacific Centre For Agricultural Engineering And Machinery, 2010. Web.
—, ‘ Sustainable agriculture key to green growth, poverty reduction – UN officials ‘, United Nations, 2011. Web.
1 United Nations, Sustainable agriculture key to green growth, poverty reduction – UN officials, UN News Centre, 2011.
2 J. D. Leaver, ‘Global food supply: a challenge for sustainable agriculture’, Nutrition Bulletin , vol. 36, 2011, pp. 416-421.
3 Leaver, p. 417.
5 Leaver, p. 418.
7 Leaver, p. 419.
8 J. N. Pretty, ‘Participatory learning for sustainable agriculture’, World Development , vol. 23, no. 8, 1995, pp. 1247-1263.
9 Chet Townsend, ‘Technology for Sustainable Agriculture’, Florida Gulf Coast University , 1998.
10 United Nations, ‘Green technology for sustainable agriculture development’, United Nations Asian And Pacific Centre For Agricultural Engineering And Machinery , 2010.
11 United Nations, p. 17.
12 J. P. Reganold, R. I. Papendick, & J. F. Parr, ‘Sustainable Agriculture’, Scientific American , 1990, pp. 112-120.
13 Regnold et al., p. 112.
14 S. S. Batie, ‘Sustainable Development: Challenges to Profession of Agricultural Economics’, American Journal of Agricultural Economics, vol. 71, no. 5, 1989, pp. 1083-1101.
15 S. Martens & G. Spaargaren, ‘The politics of sustainable consumption: the case of the Netherlands’, Sustainability: Science, Practice, & Policy , vol.1 no. 1, 2005, pp. 29-42.
16 A. Dobson, The Politics of Nature: Explorations in Green Political Theory , Psychology Press, London, 1993, p. 82.
17 C .Morris & M. Winter, ‘Integrated farming systems: the third way for European agriculture?’, Land Use Policy , vol. 16, no. 4, 1999, pp. 193–205.
- Chicago (A-D)
- Chicago (N-B)
IvyPanda. (2019, April 20). Essay on Sustainable Agriculture. https://ivypanda.com/essays/sustainable-agriculture-essay/
"Essay on Sustainable Agriculture." IvyPanda , 20 Apr. 2019, ivypanda.com/essays/sustainable-agriculture-essay/.
IvyPanda . (2019) 'Essay on Sustainable Agriculture'. 20 April.
IvyPanda . 2019. "Essay on Sustainable Agriculture." April 20, 2019. https://ivypanda.com/essays/sustainable-agriculture-essay/.
1. IvyPanda . "Essay on Sustainable Agriculture." April 20, 2019. https://ivypanda.com/essays/sustainable-agriculture-essay/.
IvyPanda . "Essay on Sustainable Agriculture." April 20, 2019. https://ivypanda.com/essays/sustainable-agriculture-essay/.
- Natural Resources, Their Classification and Impacts
- Smart Farming and Sustainable Agriculture
- Cannabis Technological Advancement in Cultivation
- Irrigation System Strategy
- Irrigation Systems in Farming
- Swidden Agriculture: Shift Farming
- Whaling in Japan: Justifiable by Culture?
- Essential Foods Price: Basics Foods and High Increase in Prices

Department of Agricultural, Food, and Resource Economics Innovation Lab for Food Security Policy, Research, Capacity and Influence
Adoption of Sustainable Agricultural Intensification Practices and their Welfare Impacts: Comparative Evidence from Malawi, Uganda and Ethiopia
May 14, 2024 - Anderson Gondwe, Lemekezani K. Chilora, Dinah Salonga, Aleksandr Michuda and Kristin Davis
Sustainable intensification practices are popular interventions for enhancing soil fertility and crop yield, and eventually improving household income and food security. Using the Living Standards Measurement Study - Integrated Surveys on Agriculture panel data from Ethiopia, Malawi, and Uganda, we conduct a multi-country comparative analysis of the adoption of sustainable intensification practices and their impacts on food and nutritional security. While most studies use the sex of the household head to define gender, we base our gender variable on decision-making: male, female, and joint households' decision-making at a farm level. We use multinomial logit, multinomial endogenous switching regression and multinomial endogenous treatment effects models to account for selection bias and endogeneity originating from both observed and unobserved heterogeneity. Our analysis shows that adoption of sustainable intensification practices is impacted household size, wealth, livestock ownership, agroecological zones, and gender decision-making at a farm level. Our econometric analysis reveals that the relationship between the adoption of sustainable intensification practices and households' food and nutritional security varies by country, confirming the importance of considering country-specific contexts and practices when designing agricultural interventions. Policymakers should consider promoting the adoption of sustainable intensification practices as they have shown to have a positive impact on food and nutritional security. Sustainable intensification practices s, along with training programs for farmers, are crucial for enhancing knowledge and resource availability to implement sustainable intensification practices and improve food and nutrition security effectively. There is a need to increase investments in agricultural research, extension services, and climate-smart agriculture.
Sustainable intensification practices, welfare, multinomial logit, multinomial endogenous switching regression and multinomial endogenous treatment effects
DOWNLOAD FILE
Tags: prci research paper
new - method size: 1 - Random key: 0, method: personalized - key: 0
You Might Also Be Interested In
STAAARS+ RFP webinar Sept 14 2022
Published on September 15, 2022
PRCI STAAARS+ Teams Presentation Video 2022
Published on July 26, 2022
Scoping Study of Agriculture Development Strategy of Nepal (ADS) (Five-year achievements)
Published on February 1, 2023
Sugarcane Production and Food Security in Uganda
Published on September 1, 2023
Institutional Arrangements Between Sugarcane Growers and Millers in Uganda and Implications for Grower Productivity and Profitability
Rwanda Natural Forest Cover Dynamics between 2015 and 2020
Published on June 19, 2023
Accessibility Questions:
For questions about accessibility and/or if you need additional accommodations for a specific document, please send an email to ANR Communications & Marketing at [email protected] .
- prci research paper,
- innovation lab for food security policy research capacity & influence
Arab States
Asia and the pacific, europe & central asia, latin america & the caribbean.
You’re using an outdated browser. Old browsers are unstable, unsafe and do not support the features of of this website. Please upgrade to continue.
Your browser does not support JavaScript. This site relies on JavaScript to structure its navigation and load images across all pages. Please enable JavaScript to continue.
What is climate change mitigation and why is it urgent?
- Share on LinkedIn
- Share on Facebook
- Share on twitter
- Share via email
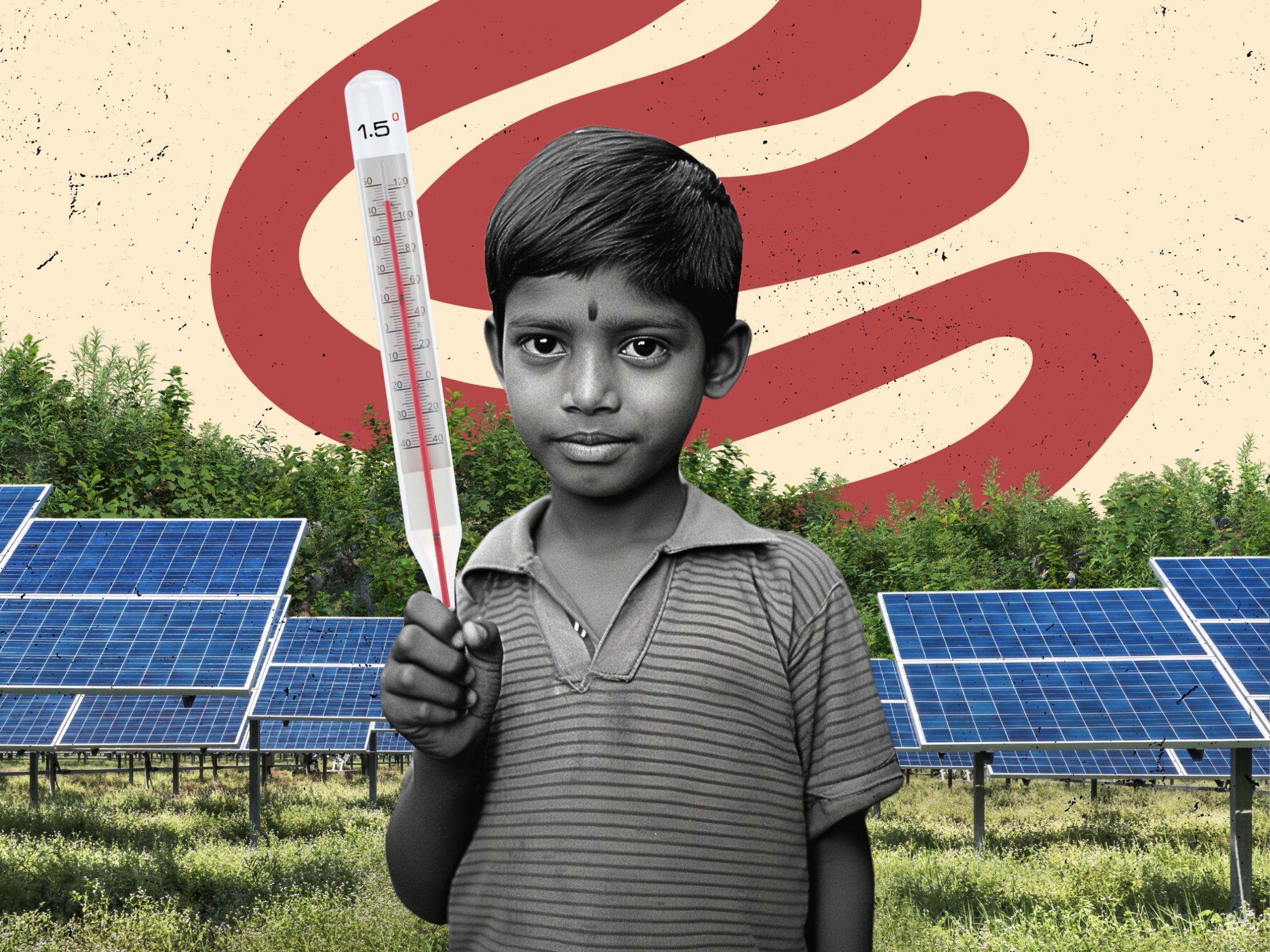
- Climate change mitigation involves actions to reduce or prevent greenhouse gas emissions from human activities.
- Mitigation efforts include transitioning to renewable energy sources, enhancing energy efficiency, adopting regenerative agricultural practices and protecting and restoring forests and critical ecosystems.
- Effective mitigation requires a whole-of-society approach and structural transformations to reduce emissions and limit global warming to 1.5°C above pre-industrial levels.
- International cooperation, for example through the Paris Agreement, is crucial in guiding and achieving global and national mitigation goals.
- Mitigation efforts face challenges such as the world's deep-rooted dependency on fossil fuels, the increased demand for new mineral resources and the difficulties in revamping our food systems.
- These challenges also offer opportunities to improve resilience and contribute to sustainable development.
What is climate change mitigation?
Climate change mitigation refers to any action taken by governments, businesses or people to reduce or prevent greenhouse gases, or to enhance carbon sinks that remove them from the atmosphere. These gases trap heat from the sun in our planet’s atmosphere, keeping it warm.
Since the industrial era began, human activities have led to the release of dangerous levels of greenhouse gases, causing global warming and climate change. However, despite unequivocal research about the impact of our activities on the planet’s climate and growing awareness of the severe danger climate change poses to our societies, greenhouse gas emissions keep rising. If we can slow down the rise in greenhouse gases, we can slow down the pace of climate change and avoid its worst consequences.
Reducing greenhouse gases can be achieved by:
- Shifting away from fossil fuels : Fossil fuels are the biggest source of greenhouse gases, so transitioning to modern renewable energy sources like solar, wind and geothermal power, and advancing sustainable modes of transportation, is crucial.
- Improving energy efficiency : Using less energy overall – in buildings, industries, public and private spaces, energy generation and transmission, and transportation – helps reduce emissions. This can be achieved by using thermal comfort standards, better insulation and energy efficient appliances, and by improving building design, energy transmission systems and vehicles.
- Changing agricultural practices : Certain farming methods release high amounts of methane and nitrous oxide, which are potent greenhouse gases. Regenerative agricultural practices – including enhancing soil health, reducing livestock-related emissions, direct seeding techniques and using cover crops – support mitigation, improve resilience and decrease the cost burden on farmers.
- The sustainable management and conservation of forests : Forests act as carbon sinks , absorbing carbon dioxide and reducing the overall concentration of greenhouse gases in the atmosphere. Measures to reduce deforestation and forest degradation are key for climate mitigation and generate multiple additional benefits such as biodiversity conservation and improved water cycles.
- Restoring and conserving critical ecosystems : In addition to forests, ecosystems such as wetlands, peatlands, and grasslands, as well as coastal biomes such as mangrove forests, also contribute significantly to carbon sequestration, while supporting biodiversity and enhancing climate resilience.
- Creating a supportive environment : Investments, policies and regulations that encourage emission reductions, such as incentives, carbon pricing and limits on emissions from key sectors are crucial to driving climate change mitigation.
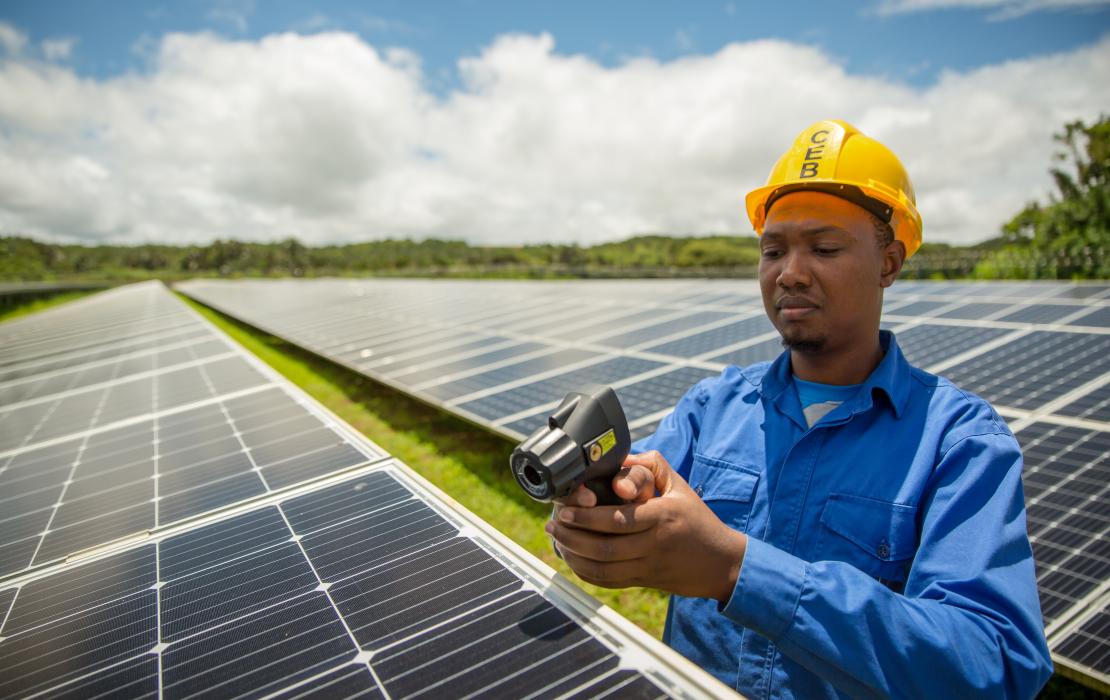
Photo: Stephane Bellerose/UNDP Mauritius
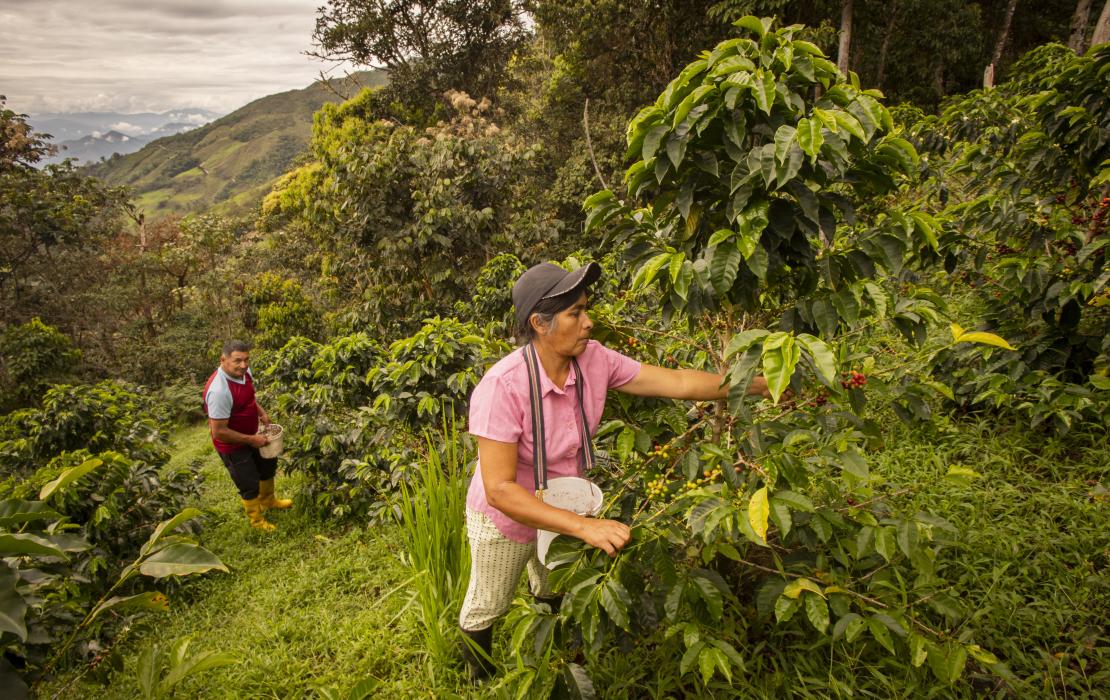
Photo: La Incre and Lizeth Jurado/PROAmazonia
What is the 1.5°C goal and why do we need to stick to it?
In 2015, 196 Parties to the UN Climate Convention in Paris adopted the Paris Agreement , a landmark international treaty, aimed at curbing global warming and addressing the effects of climate change. Its core ambition is to cap the rise in global average temperatures to well below 2°C above levels observed prior to the industrial era, while pursuing efforts to limit the increase to 1.5°C.
The 1.5°C goal is extremely important, especially for vulnerable communities already experiencing severe climate change impacts. Limiting warming below 1.5°C will translate into less extreme weather events and sea level rise, less stress on food production and water access, less biodiversity and ecosystem loss, and a lower chance of irreversible climate consequences.
To limit global warming to the critical threshold of 1.5°C, it is imperative for the world to undertake significant mitigation action. This requires a reduction in greenhouse gas emissions by 45 percent before 2030 and achieving net-zero emissions by mid-century.
What are the policy instruments that countries can use to drive mitigation?
Everyone has a role to play in climate change mitigation, from individuals adopting sustainable habits and advocating for change to governments implementing regulations, providing incentives and facilitating investments. The private sector, particularly those businesses and companies responsible for causing high emissions, should take a leading role in innovating, funding and driving climate change mitigation solutions.
International collaboration and technology transfer is also crucial given the global nature and size of the challenge. As the main platform for international cooperation on climate action, the Paris Agreement has set forth a series of responsibilities and policy tools for its signatories. One of the primary instruments for achieving the goals of the treaty is Nationally Determined Contributions (NDCs) . These are the national climate pledges that each Party is required to develop and update every five years. NDCs articulate how each country will contribute to reducing greenhouse gas emissions and enhance climate resilience. While NDCs include short- to medium-term targets, long-term low emission development strategies (LT-LEDS) are policy tools under the Paris Agreement through which countries must show how they plan to achieve carbon neutrality by mid-century. These strategies define a long-term vision that gives coherence and direction to shorter-term national climate targets.
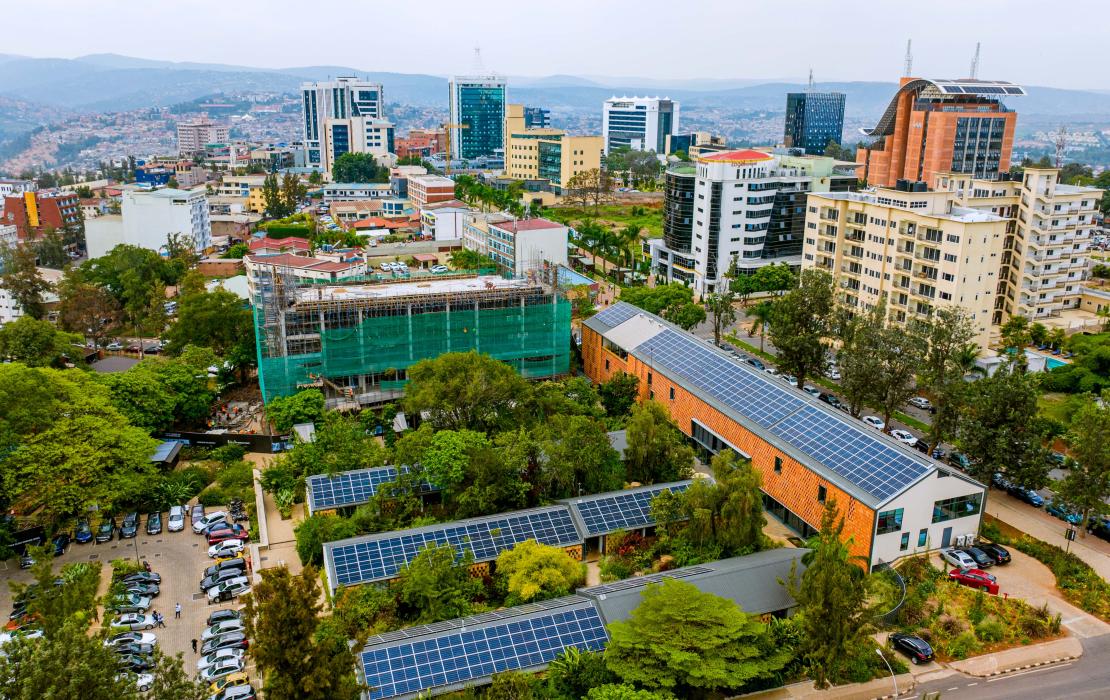
Photo: Mucyo Serge/UNDP Rwanda
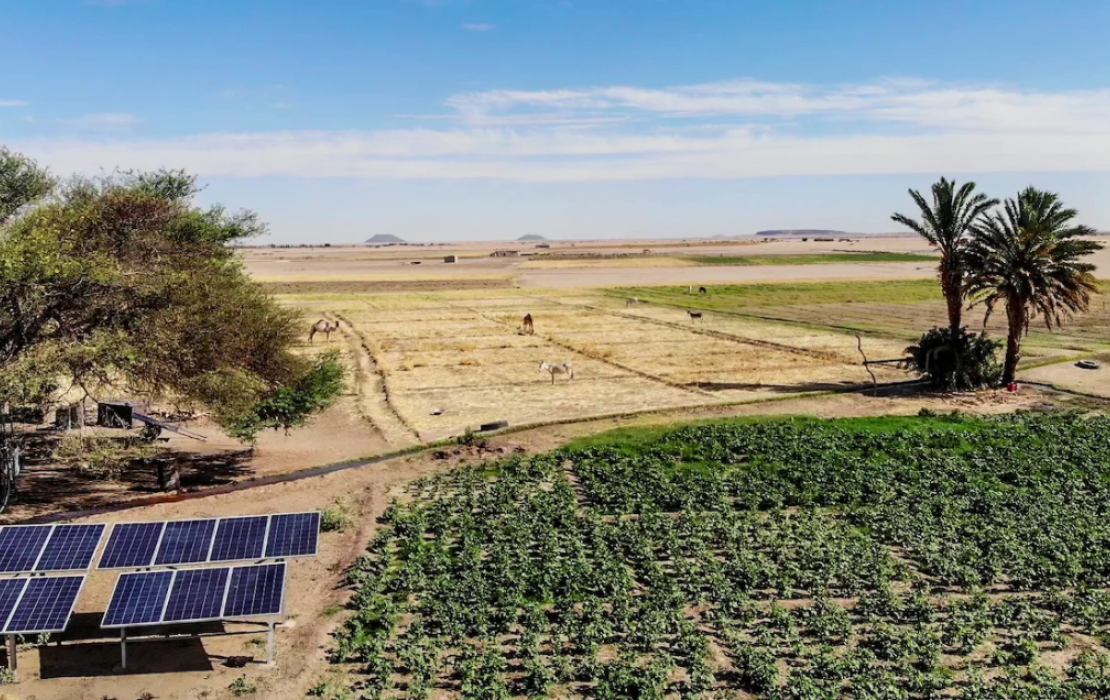
Photo: William Seal/UNDP Sudan
At the same time, the call for climate change mitigation has evolved into a call for reparative action, where high-income countries are urged to rectify past and ongoing contributions to the climate crisis. This approach reflects the UN Framework Convention on Climate Change (UNFCCC) which advocates for climate justice, recognizing the unequal historical responsibility for the climate crisis, emphasizing that wealthier countries, having profited from high-emission activities, bear a greater obligation to lead in mitigating these impacts. This includes not only reducing their own emissions, but also supporting vulnerable countries in their transition to low-emission development pathways.
Another critical aspect is ensuring a just transition for workers and communities that depend on the fossil fuel industry and its many connected industries. This process must prioritize social equity and create alternative employment opportunities as part of the shift towards renewable energy and more sustainable practices.
For emerging economies, innovation and advancements in technology have now demonstrated that robust economic growth can be achieved with clean, sustainable energy sources. By integrating renewable energy technologies such as solar, wind and geothermal power into their growth strategies, these economies can reduce their emissions, enhance energy security and create new economic opportunities and jobs. This shift not only contributes to global mitigation efforts but also sets a precedent for sustainable development.
What are some of the challenges slowing down climate change mitigation efforts?
Mitigating climate change is fraught with complexities, including the global economy's deep-rooted dependency on fossil fuels and the accompanying challenge of eliminating fossil fuel subsidies. This reliance – and the vested interests that have a stake in maintaining it – presents a significant barrier to transitioning to sustainable energy sources.
The shift towards decarbonization and renewable energy is driving increased demand for critical minerals such as copper, lithium, nickel, cobalt, and rare earth metals. Since new mining projects can take up to 15 years to yield output, mineral supply chains could become a bottleneck for decarbonization efforts. In addition, these minerals are predominantly found in a few, mostly low-income countries, which could heighten supply chain vulnerabilities and geopolitical tensions.
Furthermore, due to the significant demand for these minerals and the urgency of the energy transition, the scaled-up investment in the sector has the potential to exacerbate environmental degradation, economic and governance risks, and social inequalities, affecting the rights of Indigenous Peoples, local communities, and workers. Addressing these concerns necessitates implementing social and environmental safeguards, embracing circular economy principles, and establishing and enforcing responsible policies and regulations .
Agriculture is currently the largest driver of deforestation worldwide. A transformation in our food systems to reverse the impact that agriculture has on forests and biodiversity is undoubtedly a complex challenge. But it is also an important opportunity. The latest IPCC report highlights that adaptation and mitigation options related to land, water and food offer the greatest potential in responding to the climate crisis. Shifting to regenerative agricultural practices will not only ensure a healthy, fair and stable food supply for the world’s population, but also help to significantly reduce greenhouse gas emissions.
Photo: UNDP India
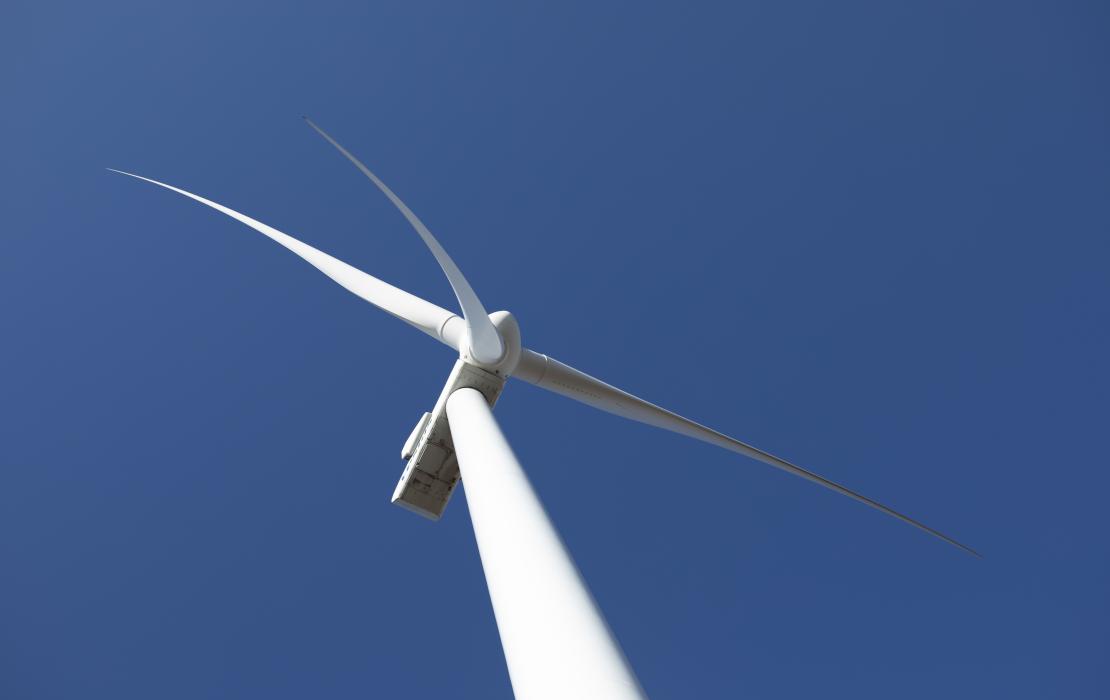
Photo: Nino Zedginidze/UNDP Georgia
What are some examples of climate change mitigation?
In Mauritius , UNDP, with funding from the Green Climate Fund, has supported the government to install battery energy storage capacity that has enabled 50 MW of intermittent renewable energy to be connected to the grid, helping to avoid 81,000 tonnes of carbon dioxide annually.
In Indonesia , UNDP has been working with the government for over a decade to support sustainable palm oil production. In 2019, the country adopted a National Action Plan on Sustainable Palm Oil, which was collaboratively developed by government, industry and civil society representatives. The plan increased the adoption of practices to minimize the adverse social and environmental effects of palm oil production and to protect forests. Since 2015, 37 million tonnes of direct greenhouse gas emissions have been avoided and 824,000 hectares of land with high conservation value have been protected.
In Moldova and Paraguay , UNDP has helped set up Green City Labs that are helping build more sustainable cities. This is achieved by implementing urban land use and mobility planning, prioritizing energy efficiency in residential buildings, introducing low-carbon public transport, implementing resource-efficient waste management, and switching to renewable energy sources.
UNDP has supported the governments of Brazil, Costa Rica, Ecuador and Indonesia to implement results-based payments through the REDD+ (Reducing emissions from deforestation and forest degradation in developing countries) framework. These include payments for environmental services and community forest management programmes that channel international climate finance resources to local actors on the ground, specifically forest communities and Indigenous Peoples.
UNDP is also supporting small island developing states like the Comoros to invest in renewable energy and sustainable infrastructure. Through the Africa Minigrids Program , solar minigrids will be installed in two priority communities, Grand Comore and Moheli, providing energy access through distributed renewable energy solutions to those hardest to reach.
And in South Africa , a UNDP initative to boost energy efficiency awareness among the general population and improve labelling standards has taken over commercial shopping malls.
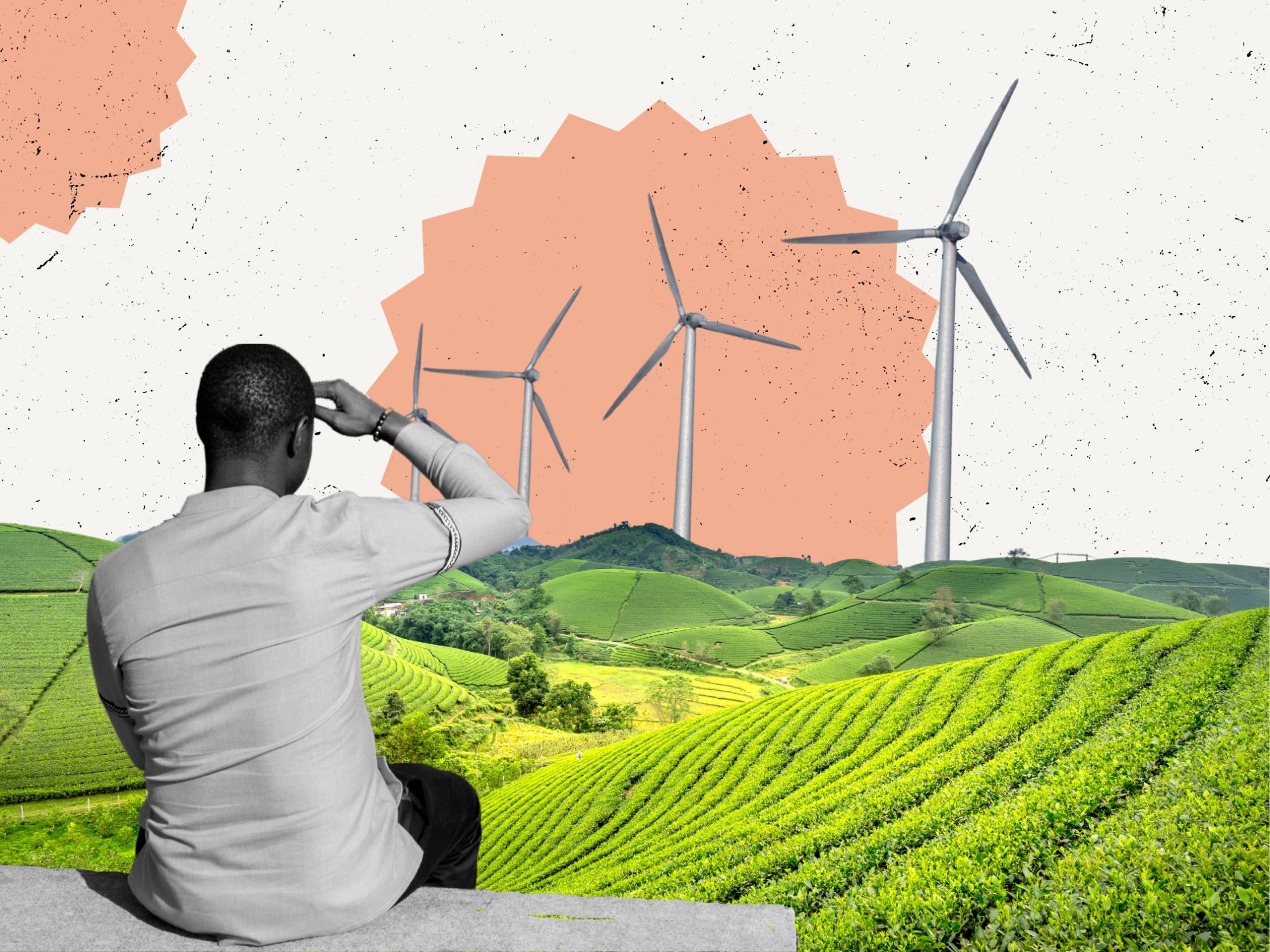
What is UNDP’s role in supporting climate change mitigation?
UNDP aims to assist countries with their climate change mitigation efforts, guiding them towards sustainable, low-carbon and climate-resilient development. This support is in line with achieving the Sustainable Development Goals (SDGs), particularly those related to affordable and clean energy (SDG7), sustainable cities and communities (SDG11), and climate action (SDG13). Specifically, UNDP’s offer of support includes developing and improving legislation and policy, standards and regulations, capacity building, knowledge dissemination, and financial mobilization for countries to pilot and scale-up mitigation solutions such as renewable energy projects, energy efficiency initiatives and sustainable land-use practices.
With financial support from the Global Environment Facility and the Green Climate Fund, UNDP has an active portfolio of 94 climate change mitigation projects in 69 countries. These initiatives are not only aimed at reducing greenhouse gas emissions, but also at contributing to sustainable and resilient development pathways.
Explore More Stories
Pacific shores, solar solutions: harnessing renewable energy in the pacific islands.
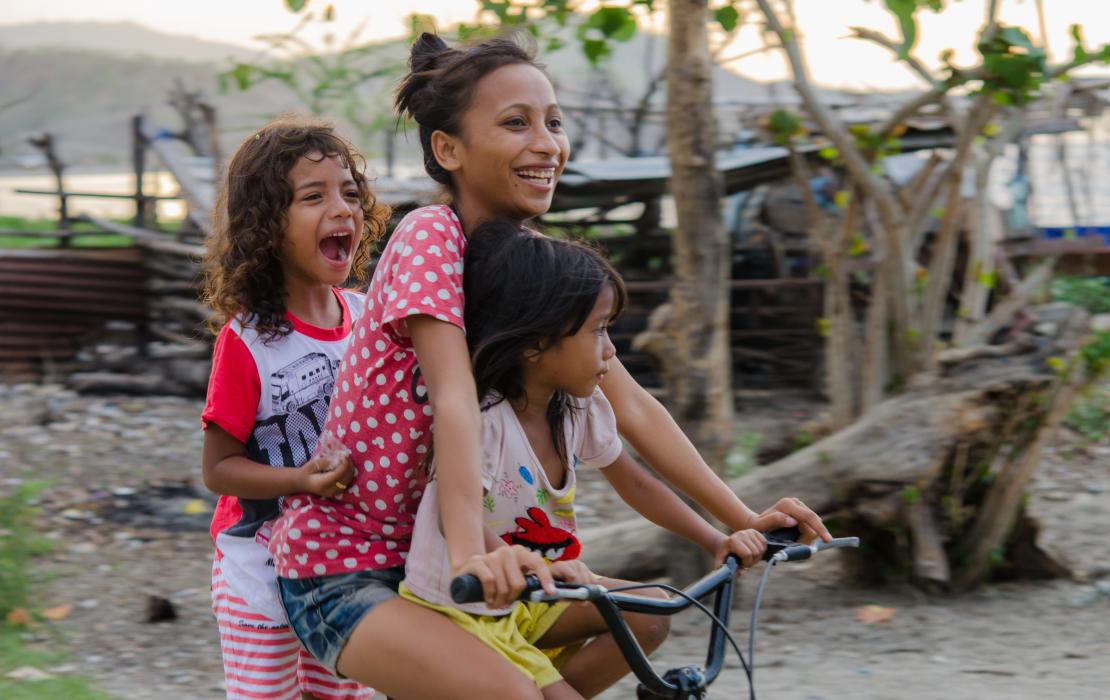
Photo: Yuichi Ishida/UNDP Timor-Leste
West Africa has great potential for solar energy. It’s time to release it.
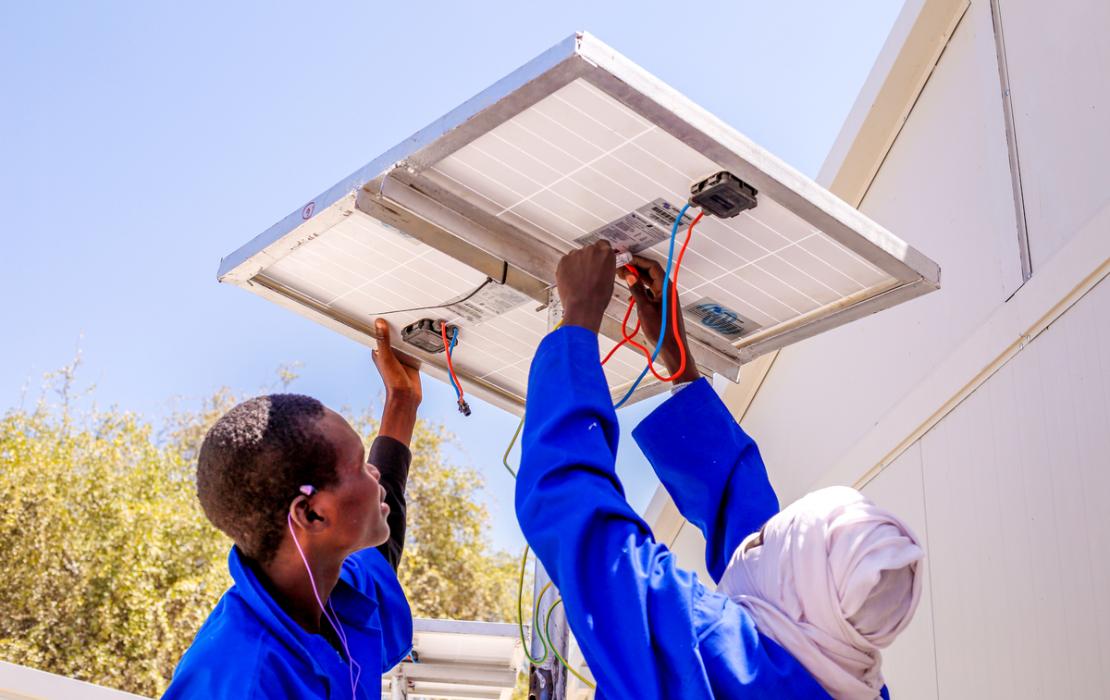
Photo: UNDP Niger
Electric vehicles are driving a greener future in Viet Nam
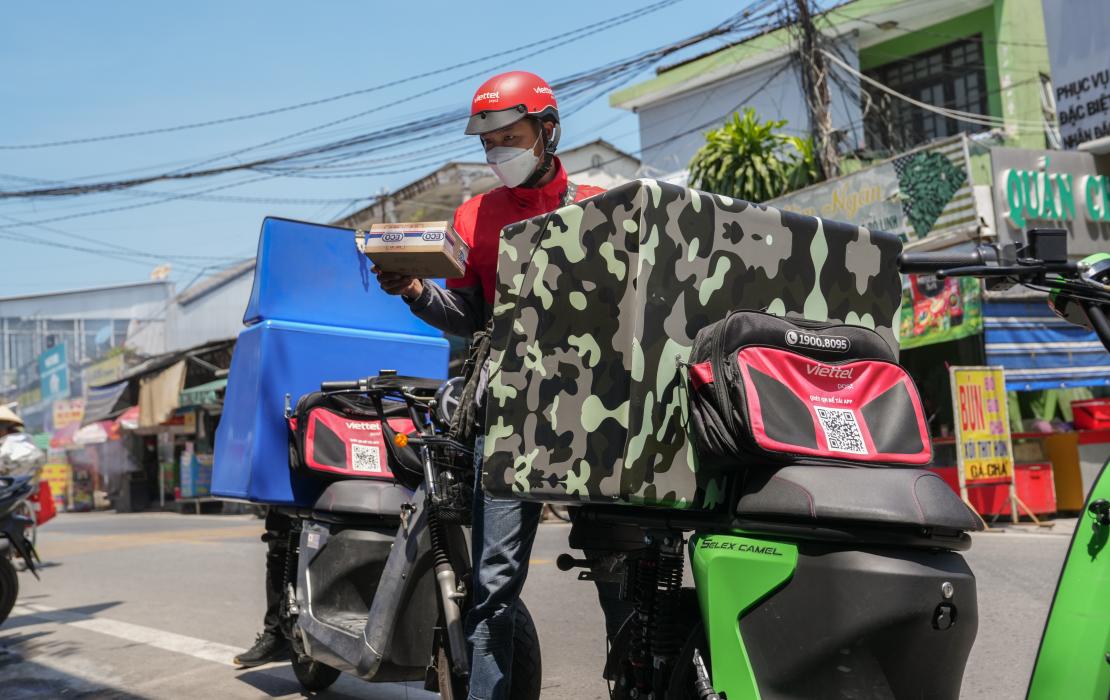
Ho Tuan Anh delivers goods with his new e-motorbike. Photo by: Phan Huong Giang/UNDP Viet Nam
Why the Western Balkans are choosing decarbonization
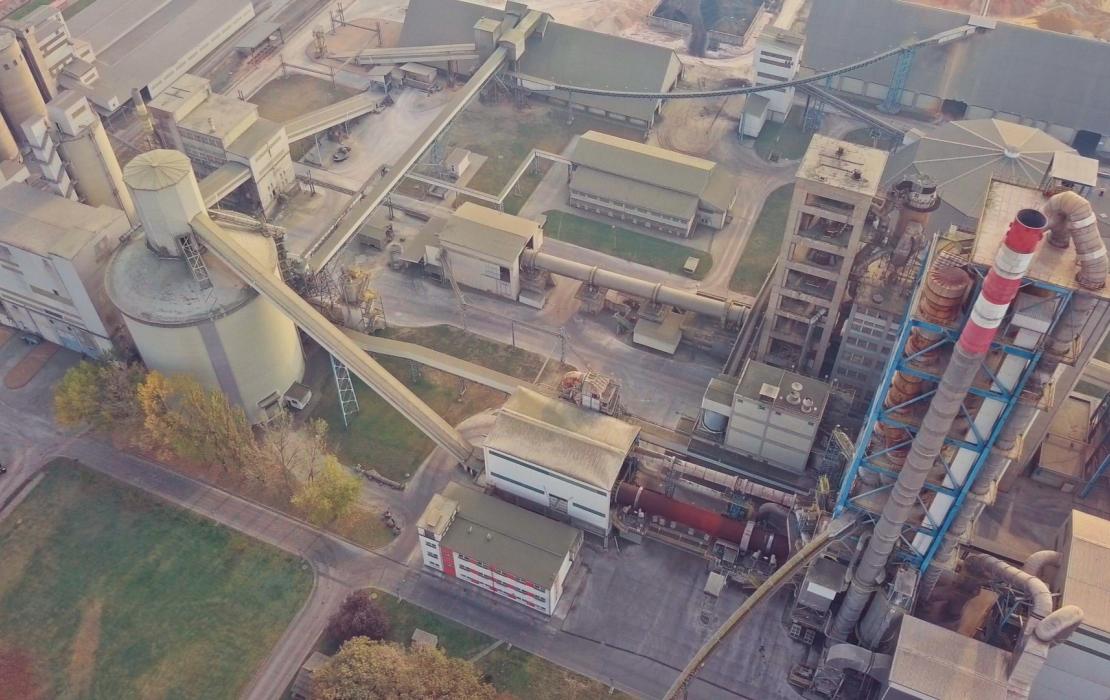
Photo: UNDP Bosnia and Herzegovina
Six lessons on how to achieve future-smart energy efficient buildings
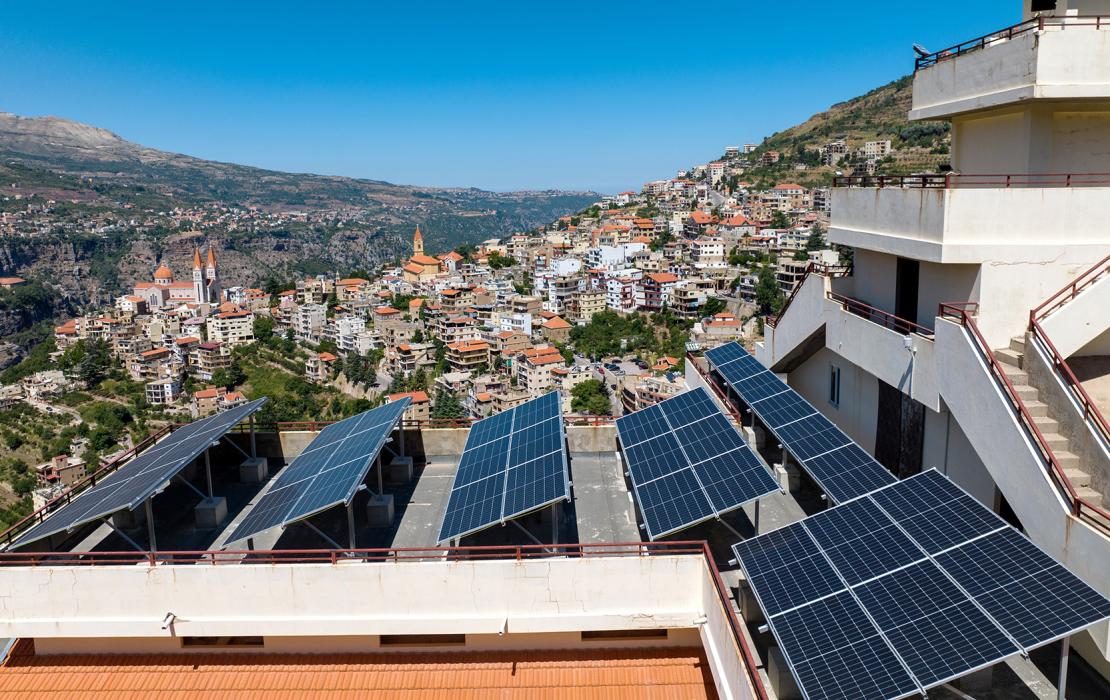
Solar photovoltaic systems on roofs in Lebanon. Photo: Fouad Choufany / UNDP Lebanon
Six ways to achieve sustainable energy for all
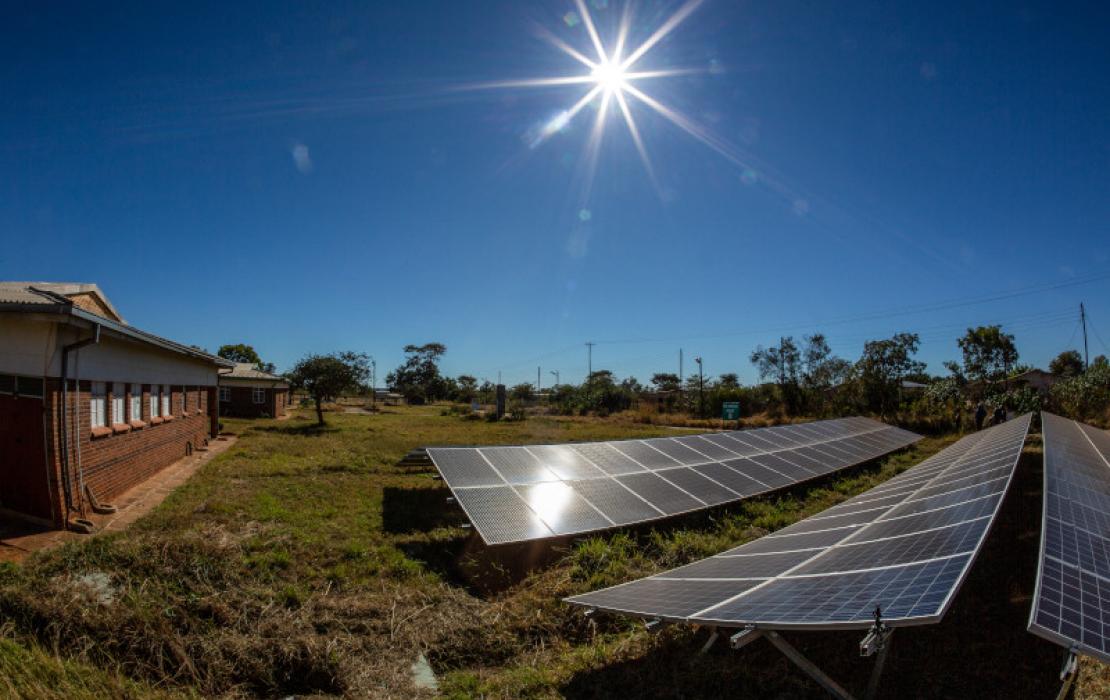
Photo: UNDP Zimbabwe
- All Research
- Funding Opportunities
- Meetings & conferences
- Protocols & Compliance
- All Teaching and Advising
- Student activities
- student honors
- Student opportunities
- All Extension and Outreach
- In the News
- All People and Departments
- Awards and honors
- Calls to nominate
- Recognition
- In Memoriam
- All Workplace
- Business Services & Payroll
- Human Resources
- Information Technology
- Position openings
- Submit a Post
College of Agricultural & Life Sciences
Department of food science offers new certificate in fermented food and beverages, amaya atucha receives sustainable agriculture grant from ncr-sare’s 2024 partnership grant program.
Amaya Atucha, associate professor and extension specialist in the Department of Plant and Agroecosystem Sciences, was awarded almost $30,000 for the project, “Establishing Honeyberry Collaborative Trials using SeedLinked to Crowdsource Cultivar Performance Data and Inform Grower Selections.”
“We seek to crowdsource performance data on honeyberry cultivars from a large network of growers, researchers and breeders. The data shared by the honeyberry community on SeedLinked will help inform grower cultivar choices and pave the way for the platform’s use with other emerging perennial crops,” says Atucha.
This grant was awarded as part of the North Central Region – Sustainable Agriculture Research and Education (NCR-SARE) 2024 Partnership Grant Program. The Partnership Grant Program is intended to foster cooperation between agriculture professionals and small groups of farmers and ranchers to catalyze on-farm research, demonstration, and education activities related to sustainable agriculture. Learn more about the NCR-SARE Partnership Grant Program online.
The focus for each of the NCR-SARE grant programs is on research and education. Funding considerations are based on how well the applicant presents the problem being addressed, the project’s relevance to sustainable agriculture in the 12-state North Central region, and how well it aligns with NCR-SARE’s goals, among other factors specific to each grant program.
NCR-SARE’s Administrative Council (AC) members decide which projects will receive SARE funds. A collection of farm and non-farm citizens, the AC includes a diverse mix of agricultural stakeholders in the region. Council members hail from regional farms and ranches, the Cooperative Extension Service, universities, federal agencies, and nonprofit organizations.
Benin: Climate Adaptation Efforts Necessary for Sustainable and Resilient Growth
COTONOU, May 13, 2024 – According to the second edition of the Benin Economic Update Report, achieving sustainable and resilient economic growth in the coming decades will depend on efforts to adapt and finance climate investments.
Titled Adapting to Climate Change for Sustainable, Resilient Economic Growth , the first part of the report analyzes recent economic developments and presents the country's medium-term outlook. It projects that annual growth will stabilize at an average of 6.2% between 2024 and 2026 (3.5% on average per capita), driven by investment and the expansion of the Glo-Djigbe industrial zone (GDIZ). The end of the gasoline subsidy in Nigeria in May 2023, supply chain bottlenecks following the closure of the border with Niger, and growing demand pressures have led to an increase in inflation to 2.8% in 2023, below the regional average of 3.7% in 2023.
Fiscal consolidation efforts were successful in 2023, owing to the adoption of innovative tax measures and spending restraint. As a result, the budget deficit was reduced to 4.1% of GDP, the lowest level since 2019, and down from 5.5% in 2022. This improvement was due to an increase in total revenues, which rose by 0.7 percentage point to 15.0% of GDP, while public spending fell by 0.6 percentage point to 19.2% of GDP. Fiscal consolidation is expected to continue in the medium term, with the budget deficit projected to decline further to 2.7% of GDP by 2026.
“Thanks to fiscal consolidation measures, Benin has been able to withstand external shocks while maintaining an appreciable economic growth trajectory. The medium-term revenue mobilization strategy will reinforce the government's domestic revenue mobilization program and make Benin's economy more resilient ,” said Felix Oppong, World Bank Senior Economist and co-author of the report .
The second part of the report looks at the vulnerabilities of the Benin economy to climate change. In line with the projections of the Country Climate and Development Report (CCDR) , the study documents that, in the absence of additional adaptation efforts, climate change could lead to increasing economic losses, with average annual GDP losses of up to 19% by 2050. By prioritizing adaptation and resilience, significant strides could be made in poverty reduction, potentially lifting almost half a million people above the poverty in contrast to a scenario where no policy action is taken.
The report calls for greater action in the agricultural sector, including the adaptation of farming practices, the restoration, and protection of forests, and investment in water resources.
“ Decisive action in the agricultural sector is essential, given its importance to Benin's economy. We need to continue diversifying agriculture in the direction of climate adaptation, promote agroforestry systems, and restore around 300,000 ha of degraded forests ,” added Manuela Ravina Da Silva, World Bank Environmental Specialist and co-author of the report.
It is also essential to address vulnerabilities in the health system, particularly those exacerbated by climate change, to strengthen the resilience of health and education services and safeguard human capital. Building resilience against urban flooding and investing in resilient transport and digital infrastructure will keep people and markets connected. Finally, Benin should prioritize partnerships with the private sector and the financing of climate investments, as the long-term benefits outweigh the costs.
“ Financing climate investments cannot rely solely on the state and its public partners; the contribution of private capital is essential. The private sector should seize the opportunities offered by climate adaptation measures to position itself as one of the engines of green growth ,” said Nestor Coffi, World Bank Country Manager for Benin.
Download the Benin Economic Update in French
This site uses cookies to optimize functionality and give you the best possible experience. If you continue to navigate this website beyond this page, cookies will be placed on your browser. To learn more about cookies, click here .
Minter receives sustainable agriculture grant
PIKETON - Logan Minter with Ohio State University, South Centers in Piketon, was awarded $49,529 for the project, "Expanding Opportunities of Season-Long Row Covers in Cucurbit Production using Native Pollinators."
"This project will engage in cooperative research to apply use of season-long row covers and native pollinators to enhance sustainability of cucurbit crops on production farms," said Minter.
This grant was awarded as part of NCR-SARE's 2024 Partnership Grant Program. The Partnership Grant Program is intended to foster cooperation between agriculture professionals and small groups of farmers and ranchers to catalyze on-farm research, demonstration, and education activities related to sustainable agriculture. Learn more about the NCR-SARE Partnership Grant Program online at http://www.northcentralsare.org/Grants/Our-Grant-Programs/Partnership-Grant-Program
The focus for each of the NCR-SARE grant programs is on research and education. Funding considerations are based on how well the applicant presents the problem being addressed, the project's relevance to sustainable agriculture in the 12-state North Central region, and how well it aligns with NCR-SARE's goals, among other factors specific to each grant program.
NCR-SARE's Administrative Council (AC) members decide which projects will receive SARE funds. A collection of farm and non-farm citizens, the AC includes a diverse mix of agricultural stakeholders in the region. Council members hail from regional farms and ranches, the Cooperative Extension Service, universities, federal agencies, and nonprofit organizations.

Budget fact sheets
The 2024–25 Federal Budget has been delivered.
Find out what has been announced in the fact sheets on this page.
Read the Portfolio Budget Statements
Agriculture Budget measures
The Budget invests in the future of Australian agriculture, fisheries and forestry through a range of new initiatives to protect and grow these industries.
- Download PDF - 445 KB
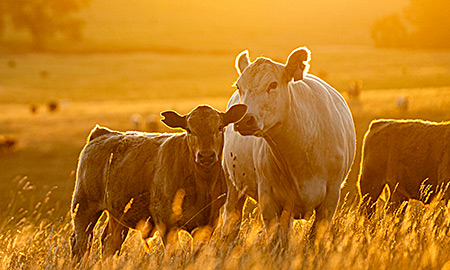
Budget 2024-25: Protecting and growing the future of Australian agriculture
- Download PDF - 322 KB
- Download Word - 526 KB
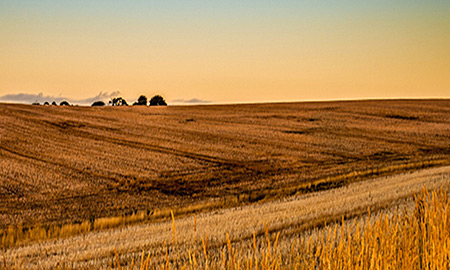
Future Drought Fund on the front foot
- Download PDF - 242 KB
- Download Word - 2.1 MB
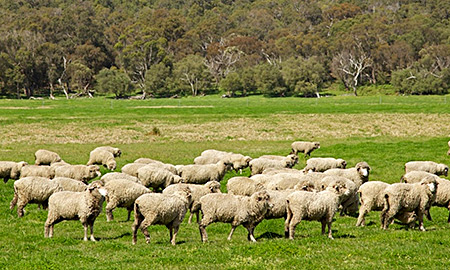
Phase Out of Live Sheep Exports by Sea – transition support
- Download PDF - 433 KB
- Download Word - 696 KB
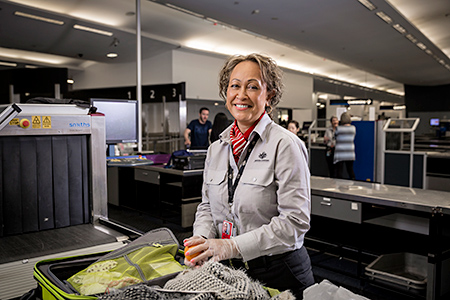
Boost to Biosecurity funding package for Western Sydney International Airport fit-out
- Download PDF - 288 KB
- Download Word - 480 KB
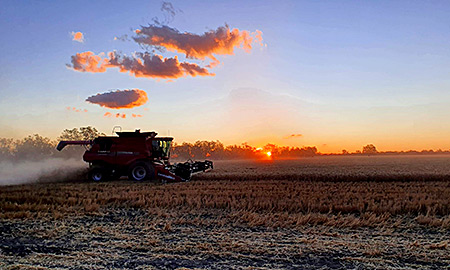
Maintaining government readiness for drought
- Download PDF - 260 KB
- Download Word - 3.4 MB
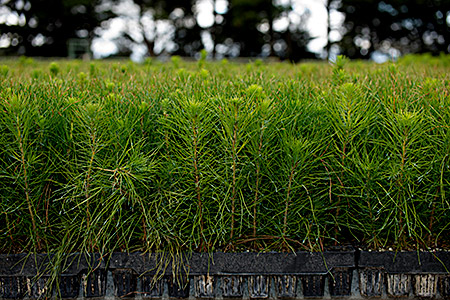
Strategy for strong forestry future
- Download PDF - 390 KB
- Download Word - 638 KB
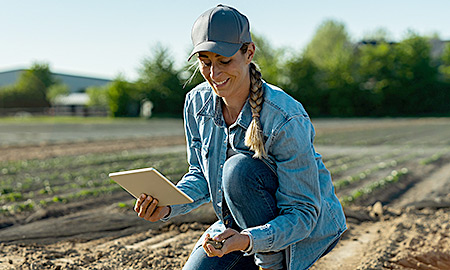
Building Australia’s agricultural workforce
- Download PDF - 286 KB
- Download Word - 418 KB
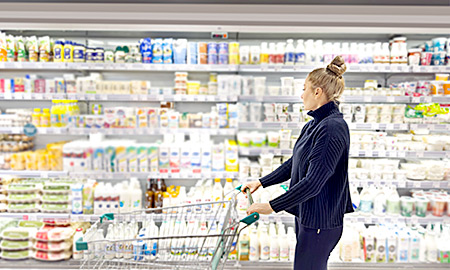
Ensuring accurate and clear plant-based and alternative protein labelling
- Download PDF - 226 KB
- Download Word - 588 KB
We aren't able to respond to your individual comments or questions. To contact us directly phone us or submit an online inquiry
Please verify that you are not a robot.
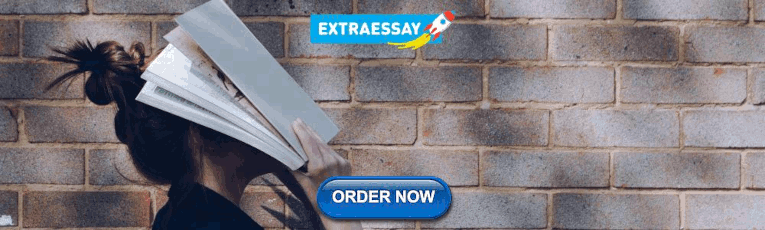
IMAGES
VIDEO
COMMENTS
Tel.: +49-4131-677-1582. Academic Editor: Marc A. Rosen. Received: 5 March 2015 / Accepted: 10 June 2015 / Published: 18 June 2015. Abstract: The idea of a sustainable agriculture has gained ...
Abstract. This chapter introduces to sustainable agriculture (SA), which is seen as a necessary way to feed and maintain/improve the quality of life of a growing world population without exacerbating environmental degradation. It starts by recalling the general importance of agriculture and showing the diversity and heterogeneity of agriculture ...
Sustainable agriculture seeks to balance human nutritional needs with the preservation of environmental quality and the economic viability of agricultural systems. Principles of resource conservation, economic resilience, social equity, and competitiveness underpin this approach. Despite its potential to address crucial issues like food security, energy sustainability, and environmental ...
The notion of food security is a global phenomenon that impinges on every human. Efforts to increase productivity and yields have historically degraded the environment and reduced biodiversity and ecosystem services, with the significant impact on the poor. Sustainable agriculture—farming in sustainable ways based on an understanding of ecosystem services—is a practical option for ...
The idea of a sustainable agriculture has gained prominence since the publication of the Brundtland Report in 1987. Yet, the concept of sustainable agriculture is very vague and ambiguous in its meaning, which renders its use and implementation extremely difficult. In this systematic review paper, we aim to advance understandings of sustainable agriculture from a social science and governance ...
Sustainable agriculture. Nature Sustainability 1 , 531 ( 2018) Cite this article. Achieving food security is possible, if we better understand the complexity of the agricultural system and re ...
Sustainable intensification improves productivity by regulating other ecosystem services and improving climate resilience (Vanlauwe et al., 2014).The introduction of alternate land use systems like silviculture, agroforestry, and hortipastoral will help diversify the available options to the farmers and lower the climate change impact on economic opportunities in the rural communities (Table ...
In sustainable agriculture, the goal is to reduce the input of external energy and to substitute non-renewable energy sources with renewable sources (e.g., solar and wind power, biofuels from ...
Sustainable agriculture, by comparison, lies at a higher and more comprehensive level. Yin: I think the concept of sustainable agriculture means that it realizes the balance of supply of agricultural products for contemporary human beings without destroying the resources for and the interests of future generations. It is the long-term stable ...
There are numerous studies and publications about sustainable agriculture. Many papers argue that sustainable agriculture is necessary, and analyze how this goal could be achieved. At the same time, studies question the sustainability of agriculture. Several obstacles, including theoretical, methodological, personal, and practical issues, hinder or slow down implementation, resulting in the so ...
Since the Green Revolution, worldwide agriculture has been characterized by a typical top-down approach. The degree of autonomy, creativity, and responsibility of farmers has been limited by the continuous external inputs of chemicals, machinery, advice, subsidies and knowledge.The issue of sustainability has brought complexity and uncertainty to this mainly linear process of innovation ...
Although the use of living microorganisms is a potent tool in the development of sustainable agriculture, it has also numerous constraints due to legal regulations [ 322 ]. As an alternative to the living microbes and cell wall polymers (CWP) of bacteria, fungi can be employed as elicitors [ 323, 324, 325 ].
Practitioners of sustainable agriculture seek to integrate three main objectives into their work: a healthy environment, economic profitability, and social and economic equity. Every person involved in the food system—growers, food processors, distributors, retailers, consumers, and waste managers—can play a role in ensuring a sustainable ...
Sustainable Agriculture. Sustainable agriculture is defined as "the efficient production of safe, high quality agricultural products, in a way that protects and improves the natural environment, the social and economic conditions of farmers, their employees and local communities, and safeguards the health and welfare of all farmed species" (Buckwell et al., 2015).
What is Sustainable Agriculture? Sustainable agriculture is one that produces abundant food without depleting the earth's resources or polluting its environment. It is agriculture that follows the principles of nature to develop systems for raising crops and livestock that are, like nature, self-sus-taining.
The term "sustainable agriculture" was defined in 1977 by the USDA as an integrated system of plant and animal production practices having a site-specific application that will, over the long term: [13] satisfy human food and fiber needs.
Sustainable agriculture represents a paradigm shift: Rather than extracting as many resources as possible, it aims to work in harmony with the natural environment, creating a self-sustaining system that will endure for generations to come. This means shifting away from our current system of intensive, animal-focused agriculture and toward a ...
Sustainable agriculture has dominated the sociological understanding of the rural world largely. Following the enthusiasm around the concept as a means of eradication of poverty and turning the economy to a "resource-efficient, low carbon Green Economy" 1. Global population, and consequently consumption has increased.
1. Theories and Methods of Agricultural Sustainable Development. Food supply is one of the important purposes of sustainable agricultural development. Gilmar et al. [ 1] used an innovative in vitro technique to increase the cultivation of micro tubers, improve the yield and quality of seeds and crops, and ensure regional food supply.
Using the Living Standards Measurement Study - Integrated Surveys on Agriculture panel data from Ethiopia, Malawi, and Uganda, we conduct a multi-country comparative analysis of the adoption of sustainable intensification practices and their impacts on food and nutritional security.
The sustainable management and conservation of forests: Forests act as carbon sinks, absorbing carbon dioxide and reducing the overall concentration of greenhouse gases in the atmosphere. Measures to reduce deforestation and forest degradation are key for climate mitigation and generate multiple additional benefits such as biodiversity ...
This grant was awarded as part of the North Central Region - Sustainable Agriculture Research and Education (NCR-SARE) 2024 Partnership Grant Program. The Partnership Grant Program is intended to foster cooperation between agriculture professionals and small groups of farmers and ranchers to catalyze on-farm research, demonstration, and ...
COTONOU, May 13, 2024 - According to the second edition of the Benin Economic Update Report, achieving sustainable and resilient economic growth in the coming decades will depend on efforts to adapt and finance climate investments.. Titled Adapting to Climate Change for Sustainable, Resilient Economic Growth, the first part of the report analyzes recent economic developments and presents the ...
The Partnership Grant Program is intended to foster cooperation between agriculture professionals and small groups of farmers and ranchers to catalyze on-farm research, demonstration, and ...
The 2024-25 Federal Budget has been delivered.Find out what has been announced in the fact sheets on this page.Read the Portfolio Budget StatementsAgriculture Budget measuresOverviewThe Budget invests in the future of Australian agriculture, fisheries and forestry through a range of new initiatives to protect and grow these industries.
To ensure sustainable agriculture in the region, a shift towards water-efficient crops is imperative. Studies focusing on crops with a balance in water management, emphasizing productivity efficiency, are crucial for adapting to the limited water environment [48,49]. The detected changes in the soil structure demand a commitment to repairing ...