Wildfire Today
News and opinion about wildland fire
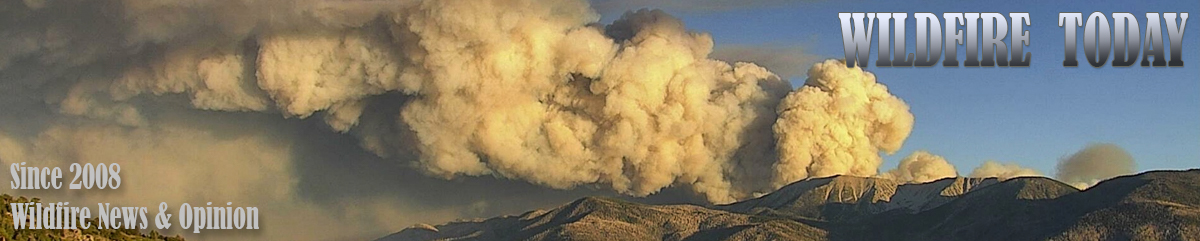
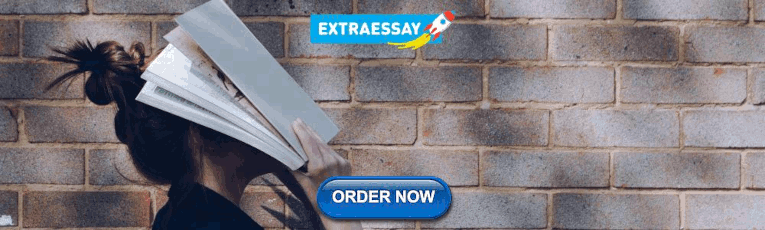
Researchers conduct detailed case study of the Camp Fire
Establish a fire progression timeline

On a brisk November morning in 2018, a fire sparked in a remote stretch of canyon in Butte County, California, a region nestled against the western slopes of the Sierra Nevada mountains. Fueled by a sea of tinder created by drought, and propelled by powerful gusts, the flames grew and traveled rapidly. In less than 24 hours, the fire had swept through the town of Paradise and other communities, leaving a charred ruin in its wake.
The Camp Fire was the costliest disaster worldwide in 2018 and, having caused 85 deaths and destroyed more than 18,000 buildings, it became both the deadliest and most destructive wildfire in California’s history, two records the fire still holds today.
What made the Camp Fire so devastating? And what lessons can we learn to prevent another disaster of this scale? Researchers at the National Institute of Standards and Technology (NIST) have begun to answer these questions by investigating the conditions leading up to the fire and meticulously reconstructing the sequence of events describing the first 24 hours of its progression. A new report containing the timeline identifies areas where more research is needed to improve life safety and reduce structural losses. It also offers a detailed look at how a large and deadly fire advances — information that will become increasingly valuable as fire seasons continue to intensify.
“Going forward, there’s no reason to believe that fire activity and severity is going to lessen anytime soon. We’re never going to get rid of wildfires, natural or human-caused. But we can learn how to live with and work together to mitigate them.” —Steven Hawks, CAL FIRE chief and report co-author
“The information we collected on the timeline is extremely powerful by itself, not only for Paradise but for other similar communities, to help them understand what they may encounter and better prepare, whether it is at a community or at the first responder level,” said NIST fire protection engineer Alexander Maranghides, who led the timeline reconstruction.
To piece together the puzzle of the Camp Fire, the team carried out discussions with 157 first responders, local officials and utilities personnel who were present during the fire. The team documented sightings of fire or smoke and efforts to fight the fire or evacuate, as well as insights into community preparedness and weather conditions.
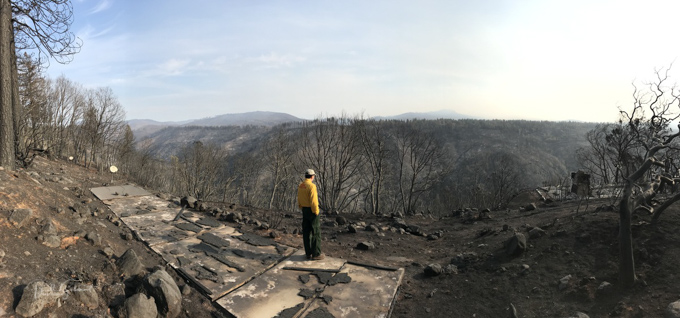
The researchers sought to back up observations made during the fire with additional data sources before adding new puzzle pieces to the timeline. With the help of the California Department of Forestry and Fire Protection (CAL FIRE), Paradise Police Department and others, the team gained access to and reviewed large data sets, including radio logs, 911 calls, dashboard and body camera recordings, and drone and satellite images. They also looked to images in social and news media to corroborate the sightings of discussion participants.
By the end of the painstaking process, the authors of the report incorporated more than 2,200 observations into the timeline, which is broken up into 15 separate segments to capture concurrent events throughout different sections of Butte County.
A Landscape Ripe for Disaster
The team’s investigation revealed several conditions throughout Butte County that, taken together, created favorable conditions for an inferno. On the day the fire broke out, wind gusts were powerful, blowing up to about 48 kilometers (30 miles) per hour, and were almost exclusively pointed southwest, toward Paradise and the smaller communities of Magalia and Concow. The 200 days of drought preceding the fire had also transformed much of the region’s lush terrain into combustible ground.
And perhaps counterintuitively, Maranghides said, the relatively large distance between the fire’s origin and the edge of Paradise (about 11 kilometers, or 7 miles) contributed to the massive, 3.2-kilometer (2-mile)-long fire front that crashed into the town.
“If a fire starts far away, upwind, then it has time to develop and expand. By the time it hits the community it is so large and so powerful that it could wipe everything out,” Maranghides said. “But if it ignites closer, the fire can be so much smaller. It takes a much smaller bite out of the community, and people may have a fighting chance.”
The report indicates that town officials in Butte County went to great lengths to prepare for fires, having cleared vegetative fuels near critical infrastructure and bolstered emergency communications in the weeks and months prior. However, dense vegetation had still accumulated throughout Paradise — a factor enhanced by the nearly 100 years the town had gone without experiencing a wildfire.
The researchers learned that, although Paradise made resources available for residents to remove trees, many did not take the city up on its offer. One participant in the reconstruction noted that residents were often attracted to the lifestyle of “living in the forest” provided by the town.
With a gamut of unfavorable conditions at play, a spark in the wilderness quickly became a raging inferno.
Breaking Down the Camp Fire’s Assault
The fire’s siege on Paradise, which ultimately destroyed 85% of the town’s buildings, began before its front line reached the city limits. Showers of burning debris were carried by the wind ahead of the main fire into town, where the embers ignited buildings and vegetation, riddling the town with dozens of smaller fires that ate up precious firefighting resources.
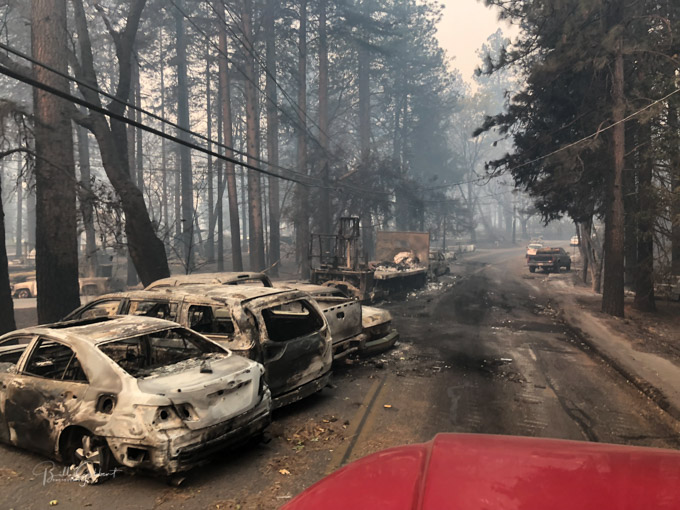
Propelling the Camp Fire’s structure-destroying spree were fires that spread within and between plots of land, or parcels, rather than from the fire front. Sources such as burning sheds, plants, vehicles and neighboring houses caused many buildings to catch fire, either through direct contact with flames or embers generated in parcels.
Paradise’s defenses quickly fell once the fire front reached town. The incident commander leading the emergency response recognized the fire’s speed and intensity and ordered his personnel to abandon all firefighting efforts just 45 minutes after the fire arrived. “Save lives, keep evacuation moving,” the incident commander said over the radio.
Although the focus of emergency response narrowed on saving lives, evacuation efforts were stifled by burnovers — life-threatening events in which residents or first responders are overrun by flames, cutting them off from escape routes. Across Paradise and Concow there were 19 burnovers at least, some of which involved downed power lines or flaming vegetation that blocked off roads, causing gridlock and putting lives in danger.
In the past, recorded burnovers were sparse, with reports attributing few or none to most fires. Hardly any have been scrutinized as heavily as the Camp Fire, however, which could partially explain the event’s high number of documented burnovers. Another critical contributor was likely Paradise’s heavily wooded nature, Maranghides said.
The abundance of burnovers during the Camp Fire may not be an isolated event, but part of a larger trend, particularly for communities where vegetative fuels have built up over many years.
“The significant activity we’ve experienced in the last few years may indicate that burnovers are becoming more frequent than they used to be,” said CAL FIRE chief Steven Hawks, a co-author of the report. “My sense is that because fires are burning so fast now, there is more potential for people to become trapped.”
Toward Fire-Safe Communities
With this report, NIST has shone light on the many aspects of the Camp Fire’s multipronged attack. Research into these threats could fill critical knowledge gaps, paving the way for science-based codes, standards and practices that could help communities outsmart fires.
What’s urgently needed, the authors write, are methods of capturing the severity of burnovers and a better understanding of how they occur in the first place. Studies in this area could make way for guidelines on reducing their likelihood and protecting evacuation routes.
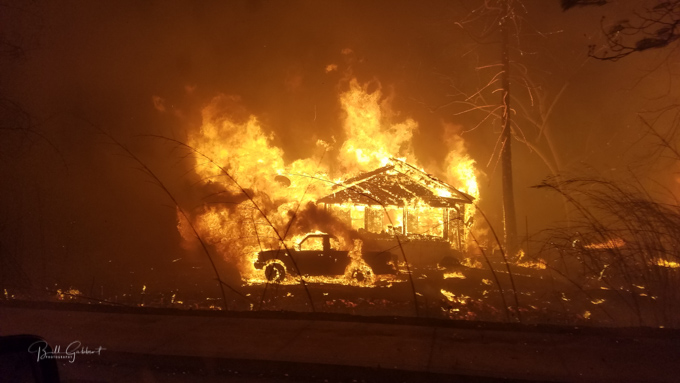
As for buildings, there are already two known options for increasing their chances of surviving a wildfire. The first is to make sure combustible items within a parcel (plants, sheds, etc.) are not too close to a structure, and the second is to increase the fire resistance of a structure’s materials. But striking a cost-effective balance between the two is difficult with the limited information on how various fuel sources threaten buildings.
“We need to improve our understanding at the parcel level because it’s the parcel-level exposures that drive the building’s survivability,” Maranghides said. “You cannot just look at the building in absence of what’s around it.”
Once researchers can put numbers to the behavior of embers and combustibles in parcels, it may become more clear what a particular building needs in terms of spacing and hardening to hold up to a wildfire.
City officials could use the report’s timeline for emergency planning as well. By having a detailed description of events such as burnovers in front of them, members of city councils or public works departments in wildfire-prone regions could evaluate their own emergency plans and potentially identify vulnerabilities.
There is currently no standard method of comparing the wildfire hazards of communities. So, although the researchers could draw individual similarities between Paradise and other communities in Northern California, they were unsure how the town compared as a whole. The team aimed to bridge this gap by developing a framework in the form of a document encouraging city officials to record specific information on fuels, population, emergency notifications and other aspects of the community.
If adopted and employed statewide in California and in other wildfire-prone areas, the framework, which appears in the report, could reveal areas most at risk and worthy of attention and resources, Maranghides said.
In the hands of first responders, the new report could become valuable training material. Using data on how quickly and intensely the fire grew, commanders could build tabletop exercises to practice deploying firefighting resources to counter its spread and save lives.
An event on the scale of the Camp Fire makes it clear that action is needed at all levels to protect communities from wildfires, Hawks said. And that need is perhaps more urgent now than ever.
“Going forward, there’s no reason to believe that fire activity and severity is going to lessen anytime soon,” Hawks said. “We’re never going to get rid of wildfires, natural or human-caused. But we can learn how to live with and work together to mitigate them.”
The full report is now available, along with several maps portraying the fire spread. The timeline of fire progression will form the basis for subsequent reports on evacuation and emergency response during the Camp Fire that the NIST team plans to publish in the coming months.
Thanks and a tip of the hat go out to Paula and Jack.
Typos, let us know HERE , and specify which article. Please read the commenting rules before you post a comment.
Share this:
Author: Bill Gabbert
After working full time in wildland fire for 33 years, he continues to learn, and strives to be a Student of Fire. View all posts by Bill Gabbert
One thought on “Researchers conduct detailed case study of the Camp Fire”
My wife and I stayed and fought the fire for 12 hours. It wasn’t a rash or emotional decision. We had prepared for a fire by extending our defensible space for four years before the fire. We had seven hose bibs, all with hoses on them. We ended up by combining all the hoses to make two 200-foot hoses AND SAVED 4 HOUSES. My wife literally put out the fire that started on the house next door. I have lived in my house for 37 years. I first lived here in 1955 and my wife has lived here continuously since 1968. When it comes to fire, we know what works and what doesn’t. We have lots of pictures and videos of before and during the fire. Some show exactly what you talked about. Mainly, structures and objects that catch on fire and cause the next house to catch on fire. We would love to have a way of showing the people in charge, videos showing what caused the fire to spread from house to house and some simple building code changes that could have saved many houses.
Comments are closed.
- Skip to main content
- Keyboard shortcuts for audio player
Wildfire smoke contributes to thousands of deaths each year in the U.S.
Alejandra Borunda

Wildfire smoke covered huge swaths of the U.S. in 2023, including places like New York City, where it has historically been uncommon. New research shows the health costs of breathing in wildfire smoke can be high. David Dee Delgado/Getty Images hide caption
Wildfire smoke covered huge swaths of the U.S. in 2023, including places like New York City, where it has historically been uncommon. New research shows the health costs of breathing in wildfire smoke can be high.
New research shows that the health consequences of wildfire smoke exposure stretch well beyond the smoky days themselves, contributing to nearly 16,000 deaths each year across the U.S., according to a National Bureau of Economic Research (NBER) analysis released in April. The analysis warns that number could grow to nearly 30,000 deaths a year by the middle of the century as human-driven climate change increases the likelihood of large, intense, smoke-spewing wildfires in the Western U.S. and beyond.
"This really points to the urgency of the problem," says Minhao Qiu, a researcher at Stanford University and the lead author. "Based on our results, this should be one of the policy priorities, or the climate policy priority, of the U.S., to figure out how to reduce this number."
Another analysis , led by researchers from Yale University, finds that the human death toll every year from wildfire smoke could already be near 30,000 people in the U.S. Deaths from cardiovascular disease, respiratory problems, kidney disease, and mental health issues all rise in the days and weeks after smoke exposure.
Together, the studies point to an underappreciated threat to public health, says Yiqun Ma, a researcher at Yale and an author of the second study.
"It's a call to action," she says—outlining the real, and significant, human stakes of failing to rein in further human-caused climate change.
How bad is smoke for health?
Wildfire smoke is rarely listed as a cause of death on people's death certificates. But research has shown that tiny particles present in smoke worsen many different health problems. These particles penetrate deep into people's lungs and can cross into the bloodstream or even into the brain. Repeated exposures, or high-concentration exposures, can supercharge other health problems, from heart and kidney disease to hastening the onset of dementia symptoms. In some cases, the stress from wildfire smoke is so great that some people die.
Because the harm from wildfire smoke can accumulate and isn't always immediately obvious, the long-term risks from wildfire smoke exposure have gone underappreciated.
"It's not obvious, necessarily, if you're looking at any individual case," says Sam Heft-Neal, an environmental economist at Stanford and an author of the NBER study. Stepping back and looking at the data statistically makes the picture much clearer, he says: smoke is a big problem that is contributing to thousands of deaths already in the U.S.
Physician and researcher Juan Aguilera, now at the University of Texas School of Public Health in El Paso, has studied the impacts of air pollution on his patients' health. He had just moved to the San Francisco Bay Area in 2020 when the smoke descended. "The 2020 wildfires brought a lot of perspective to people living in the Bay Area," he says.
Aguilera and colleagues sampled people's blood before and during the smoke event. They found markers of stressed immune systems and inflammation , signs that people were heavily impacted during the smoke. "As scientists, we do understand that things like chronic inflammation, chronic stress, lead to chronic conditions that are often related to mortality," he says.
The exact mechanisms by which smoke impacts people's health are still being unraveled. Some evidence suggests that wildfire smoke is more harmful than other tiny particles, like pollution from fossil fuel combustion or fine dust. It's likely more harmful smoke is produced when wildfires burn through urban areas, where everything from houses full of insulation to car batteries, and metal are torched.
Aguilera compares the risk of inhaling wildfire smoke to smoking cigarettes. "Being in a wildfire-prone area, it seems something equivalent to smoking like one pack a day, or 10 packs a week," says Aguilera.
Big problems, big impacts
Despite the growing understanding of the health risks from wildfire smoke, the costs have not been factored into most policy decisions, says Susan Anenberg, a public health and pollution expert at George Washington University.
The new research adds to a body of work "showing that wildfire smoke is one of the largest public health consequences of climate change," Anenberg says.
By 2050, the overall annual economic cost credited to lives lost from wildfire smoke could reach $240 billion, according to the NBER analysis. That is larger than previous estimates of all climate-related damages combined—including direct costs related to wildfire and tropical cyclone damages.
"Our estimates of the damages of climate change are undercounting the true effects," Anenberg says.
The NBER analysis used a suite of different computer models, trained on fire observations from 2000 to 2021, to figure out the relationship between fire activity and how much smoke was produced. The researchers then linked that smoke to weather patterns, letting them see how the smoke spread and drifted into different parts of the U.S. at various times. They linked those maps of smoke pollution to county-level death records across the country from 2006 to 2019 to see how deaths changed when the overall exposure to wildfire smoke went up or down.
In years like 2020, some northern California counties were exposed to double their normal pollution load for the year. In conditions like those, the total number of deaths increased by almost 6%. But even small increases in smoke exposure averaged out over the year, push mortality up. "Our findings are consistent with a host of recent work suggesting there is no safe level of air pollution exposure," the study authors write.
Those same models also let them look further into a climate-changed future. Even with aggressive climate action in coming decades, wildfire activity is forecast to grow—and with it, smoke exposure. By the middle of the century, models suggest people across the U.S. will likely experience two to three times as much smoke as they did before 2020. Smoke-related deaths could rise by at least 8,000 people every year. With less aggressive climate action, the number of deaths could be even higher.
"Not to be an alarmist, but the results are staggering," says Aguilera. "They paint a difficult picture for years to come."
The Yale study, led by Ma, uses a similar strategy to estimate the impact of smoke on deaths across the country. But the researchers also looked at the recorded causes of death. Even at very low concentrations, smoke was associated with a higher frequency of deaths related to heart disease. They also saw upticks in the number of deaths related to mental health, endocrine problems, and even diabetes.
- Original research
- Open access
- Published: 25 August 2021
Large California wildfires: 2020 fires in historical context
- Jon E. Keeley ORCID: orcid.org/0000-0002-4564-6521 1 , 2 &
- Alexandra D. Syphard 3
Fire Ecology volume 17 , Article number: 22 ( 2021 ) Cite this article
28k Accesses
74 Citations
33 Altmetric
Metrics details
California in the year 2020 experienced a record breaking number of large fires. Here, we place this and other recent years in a historical context by examining records of large fire events in the state back to 1860. Since drought is commonly associated with large fire events, we investigated the relationship of large fire events to droughts over this 160 years period.
This study shows that extreme fire events such as seen in 2020 are not unknown historically, and what stands out as distinctly new is the increased number of large fires (defined here as > 10,000 ha) in the last couple years, most prominently in 2020. Nevertheless, there have been other periods with even greater numbers of large fires, e.g., 1929 had the second greatest number of large fires. In fact, the 1920’s decade stands out as one with many large fires.
Conclusions
In the last decade, there have been several years with exceptionally large fires. Earlier records show fires of similar size in the nineteenth and early twentieth century. Lengthy droughts, as measured by the Palmer Drought Severity Index (PDSI), were associated with the peaks in large fires in both the 1920s and the early twenty-first century.
Antecedentes
En el año 2020, California experimentó un récord al quebrar el número de grandes incendios. Aquí situamos a éste y otros años en un contexto histórico mediante el examen de registros de incendios en el estado desde 1860. Dado que la sequía es frecuentemente asociada a grandes eventos de incendios, investigamos la relación entre grandes incendios y sequías en este período de 160 años.
Este estudio mostró que eventos extremos como el visto en 2020 no son históricamente desconocidos, y lo que se muestra como distintivamente nuevo es el incremento en el número de grandes incendios (definidos aquí como > 10.000 ha) en el último par de años, y más prominentemente en 2020. Sin embargo, ha habido otros períodos con aún mayores números de incendios (i.e. en 1929 hubo mayor número de incendios que en cualquier otro año del registro). De hecho, la década de 1920, fue una de las que presentó mayor número de grandes incendios.
Conclusiones
En la última década ha habido muchos años con incendios excepcionalmente grandes. Antiguos registros muestran incendios de tamaño similar tanto en el siglo 19 como en el siglo 20. Sequías prolongadas, medidas mediante el Índice de Sequías Severas de Palmer (PDSI), fueron asociadas con los picos de grandes incendios tanto en el siglo 20 como en el 21.
Introduction
The western US has a long history of large wildfires, and there is evidence that these were not uncommon on pre-EuroAmerican landscapes (Keane et al. 2008 ; Baker 2014 ; Lombardo et al. 2009 ). One of the biggest historical events was the 1910 “Big Blowup,” which reached epic proportions and was an important impetus for fire suppression policy (Diaz and Swetnam 2013 ). California in particular has had a history of massive wildfires such as the 100,000 ha 1889 Santiago Canyon Fire in Orange County or the similarly large 1932 Matilija Fire or 1970 Laguna Fire (Keeley and Zedler 2009 ).
While large fires are known in the historical record, in the first few decades of the twenty-first century, the pace of these events has greatly accelerated (Keeley and Syphard 2019 ). In the last decade, the state has experienced a substantial number of fires ranging from 10,000 ha to more than 100,000 ha, and these have caused massive losses of lives and property. The largest fires on record were recorded in 2018 and then were replaced with even larger fires in 2020, although some of these were the result of multiple fires that coalesced into fire complexes of massive size.
Causes for these fires are multiple, but climate change has been implicated as a critical factor (Williams et al. 2019 ; Abatzoglou et al. 2019 ). Historically, drought has often been invoked as a driver of large fires (Keeley and Zedler 2009 ; Diaz and Swetnam 2013 ), and California has experienced an unprecedented drought in the last decade (Robeson 2015 ). However, factors such as management impacts on forest structure and fuel accumulation, made worse by the recent drought, are critically important in some ecosystems (Stephens et al. 2018 ).
To put these recent fires in a historical context, we have investigated the history of large wildfires in California. “Large” fires is an arbitrary designation, e.g., Nagy et al. ( 2018 ) considered it to be 1000 ha or more. Our focus, however, is on those fires that made 2020 particularly noteworthy; so we define large fires as those in the top 1–2% of all fires, which is approximated by fires > 10,000 ha. In addition, we have examined the relationship of large fires to drought.
The database of fires > 10,000 ha was assembled from diverse sources. From 1950 to the present, the State of California Fire and Resource Assessment Program (FRAP) fire history database was relatively complete, but less so prior to 1950 (Syphard and Keeley 2016 ; Miller et al. 2021 ). In California, US Forest Service (USFS) annual reports provide statistics on fires by ignition source and area burned back to 1910 and Cal Fire back to 1919 (Keeley and Syphard 2017 ), and although these reports focused on annual summaries, they often provided descriptions of very large fires. A rich but under-utilized historical record for early years was the exhaustive compilation of fires in a diversity of documents from 1848 to 1937, assembled by a USFS project and brought to our attention by Cermak’s ( 2005 ) USFS report on Region 5 fire history. This source presents all documents (including agency reports and newspaper reports on fire, vegetation, timber harvesting and Native Americans) for all counties in the state and comprises 69 bound volumes (USDA Forest Service 1939-1941 ). We utilized these documents where they presented data on fire size, either an estimate of acres burned or dimensions of the burned area. We did not include fire reports that lacked a clear indication of area burned; e.g., the 1848 fire described in the region of Eldorado County referred to an immense plain on fire and all the hills blackened for an extensive distance (USDA Forest Service 1939-1941 ), but lacked more precise measures.
Other sources included the following: Barrett ( 1935 ), based on USFS records and personal experiences as well as “early-day diaries, historical works, magazines and newspapers.” Greenlee and Moldenke ( 1982 ) included fire records from state and federal agencies as well as library and museum archives. Morford ( 1984 ) was based on unpublished USFS records accumulated during the author’s 41 years in that agency. Keeley and Zedler ( 2009 ) was based on records retrieved from the California State Archives and State Library. Cal Fire ( 2020 ) data, not part of the FRAP database, included agency records of individual fire reports (not available to the public but searchable by the State Fire Marshall Kate Dobrinsky). In a few cases, the same fire was reported by more than one source, sometimes with different sizes; when this occurred after 1950, we used the FRAP data and before that either Cermak ( 2005 ) or Barrett ( 1935 ) over other sources.
Reliability of these data sources is an important question to address. Stephens ( 2005 ) contended that USFS data before 1940 were unreliable, an assertion based on Mitchell ( 1947 ); but Mitchell ( 1947 ) provided no evidence that early data were inaccurate, only that many states lacked early records. Mitchell ( 1947 ) was considering availability of state and federal data for the entire USA; however, California has far better historical records at both the state and federal archives than much of the USA (Keeley and Syphard 2017 ). USFS records for California were reported annually for all forests beginning in 1910 and for state protected lands by Cal Fire back to 1919. The latter agency had by 1920 several hundred fire wardens strategically placed throughout the state and each warden was held to a strict standard of reporting all fires in their jurisdiction.
Before 1910, data on fires was dependent on unpublished reports available in state and federal archives, observations published in books, data given in newspaper accounts of fire events, and estimates from fire-scar chronology studies. It was suggested by Goforth and Minnich ( 2007 ) that early newspaper reports were exaggerations and represented “yellow journalism,” a pejorative term that connoted unethical journalism. This was based on what they considered sensational headlines, but comparison of nineteenth century with more recent newspaper headlines provides no basis for this conclusion (Keeley and Zedler 2009 ). As a journalist colleague suggested, “a century-old newspaper story is not a precise source …[but] is the first draft of history and a valuable source of first person account from long past events.” Such information qualifies as scientific evidence, which is defined as evidence that serves to either support or counter a scientific theory or hypothesis, is empirical, and interpretable in accordance with scientific method. The data we present falls within these bounds and that includes newspaper reports as we used data on fire size in terms of acres or dimensions of burned landscape reported. Recently Howard et al. ( 2021 ) demonstrated that fire-scar records match newspaper accounts in the eastern US. To address the issue of how close newspaper accounts used in this study come to accurately depicting fire size, we have compared fires reported in published sources with newspapers where available. We of course appreciate that early accounts lacked the precise technology available today for outlining fire perimeters; however, this lack of precision does not necessarily translate into less accurate accounts and applies to both newspapers as well as state and federal agencies.
Data were presented for the state and by NOAA divisions North Coast (1), North Interior (2), Central Coast (4), Sierra Nevada (5), and South Coast (6). These are the five most fire-prone divisions of NOAA’s National Climatic Data Center categories, defined as climatically homogenous areas (Guttman and Quayle 1996 ). There of course are other systems that may be useful for comparisons, dependent on the need. For example, the Bailey Ecoregions (Bailey 1980 ), which separates regions by vegetation type, might be thought preferable, but, for our purposes, there is no necessary advantage as large fires usually burn across a mosaic of different vegetation types. A system that might provide a better presentation would be the recently described Fire Regime Ecoregions (Syphard and Keeley 2020 ). However, despite limitations to the NOAA divisions (e.g., Vose et al. 2014 ), it is preferable due to the availability of historical annual data on the Palmer Drought Severity index calculated by NOAA divisions.
Palmer Drought Severity Index (PDSI) was recorded for each year from two sources. From 1895 to 2020, PDSI was the annual mean from NOAA ( 2020a ), and for years prior to 1895, summer PDSI was reconstructed from tree-ring studies (Cook et al. 1999 ). Statistical analysis and graphical presentation were conducted with Systat software (ver. 13.0, Systat Software, Inc., San Jose, CA, http://www.systat.com/ ).
Since some of the fires came from data reported in newspapers, not a typical scientific data base, we did an initial investigation comparing FRAP reported fire size with size reported in newspaper reports. This was not an exhaustive study since FRAP data before 1950 presents relatively few fires by date or fire name making it difficult to match up fires with newspaper reports; however, we found half a dozen potential comparisons (Table 1 ). As to be expected these different reports are not identical in fire size, however, they were quite similar; sometimes, newspapers over reported area burn but other times under reported, although most importantly, they were of similar magnitude as those in the FRAP database. Data sources varied over time (Table 2 ); from 1950 to the present, large fires were all recorded in the FRAP database. Prior to that year, sources were mostly from USFS ( 1939-1941 ).
Fire size of all fires over 10,000 ha during the last 160 years are shown in Fig. 1 a. Exceptionally large fires followed a bimodal pattern with peaks in the nineteenth century and again in the twenty-first century, separated by a low point in the 1950s. From 1860 to 1950, there was a significant decrease in large fire size followed by a significant increase in the second half of the record. Although the trends were highly significant, the great year to year variation in size of large fires, gave low r 2 values, indicating limited ability to predict fire size for any given year.
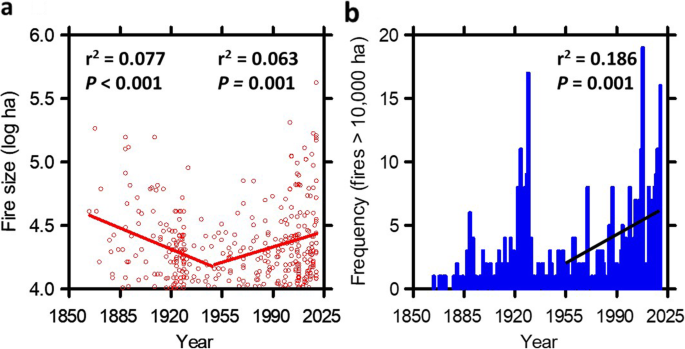
a Fire size for large fires from 1860 to 2020. b Frequency of large fires over this same time period
To illustrate the temporal distribution of record-breaking fires, we picked the top 3% ( n = 12) of all fires based on size, and these are shown in (Table 3 ). Not surprisingly, 5 occurred in the year 2020; however, four occurred in the nineteenth century.
The data presented in this paper greatly expands our understanding of the history of large fires in California. To date, our dependence has been on the FRAP database and they clearly acknowledge their records are for fires from 1950 to the present, and this is borne out by our analysis (Table 2 ), but the records presented here extend the fire history back nearly a century. Over the period from 1860 to the present, yearly frequency of fires over 10,000 ha exhibited several prominent peaks (Fig. 1 b). A few peak years occurred in the 1920s, with one of the highest frequencies recorded throughout the entire 160 year record in 1929. There were also peaks in 2007 and 2008 and again in 2018 and 2020.
Through time, the distribution of fire size varied between NOAA divisions (Fig. 2 ). The North Interior (2), Sierra Nevada (5), and South Coast (6) divisions all exhibited a significant decline in fire size from the nineteenth century till 1950. Although all the regions exhibited the largest fires in the last decade, only in the Central Coast (4) was this significant for the years 1950–2020.
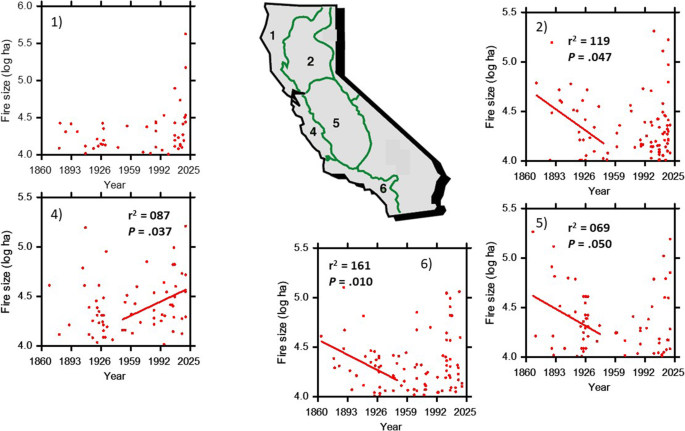
Large fires within NOAA Divisions. Statistics are presented for significant trends
Frequency of fires over 10,000 ha are presented by decade for each of the five divisions (Fig. 3 ). Consistent with the statewide pattern (Fig. 1 b), all showed a spike in number of large fires in the 1920s and again after 2000. The 1920s peak was particularly prominent in the Sierra Nevada (5) and South Coast. Also, for the Central Coast and Central Sierra Nevada regions, the number of fires in the 1920s was higher than that for recent years. For the years 1860 to 1949 and for the years 1950 to 2020 separately, there was no significant change in frequency over time.

Decadal frequency of large fires within NOAA Divisions. Note the decade 2020 is represented by a single year.
One aspect of climate over the entire period is captured by the PDSI, a drought index that includes patterns of both precipitation and temperature. There have been several periods of drought over the past 160 years, the most severe being in the decades 1920-1930 and 1990-2020 (Fig. 4 a). These periods also correlate with periods of large amounts of area burned by large fires (Fig. 4 b). Bivariate regression analysis showed that over the period from 1860 to 2020, there was a significant relationship between PDSI and area burned (adj r 2 = 0.429, P = 0.003).
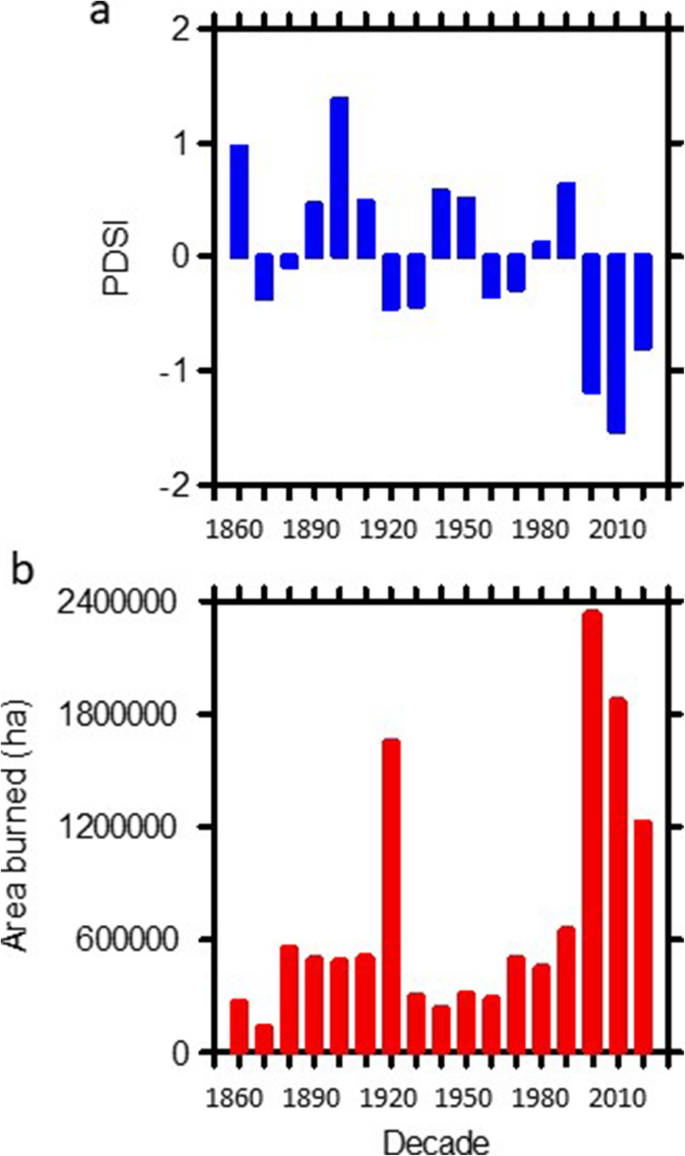
a PDSI for the decades from 1860 to 2020. b Area burned by fires > 10,000 ha for the decades from 1860 to 2020. Note the decade 2020 is represented by a single year
Clearly, 2020 was a phenomenal fire year in California for record breaking large fires. However, this study shows that such extreme fire events are not unknown historically, and what stands out as distinctly new is the increased number of large fires (defined here as > 10,000 ha) in the last couple of years, most prominently in 2020. Given that historically we have seen years with even greater number of large fire events, e.g., 1929, a comprehensive evaluation of the factors leading up to large fire event years is clearly needed.
The largest fire in recorded history for the state is the 2020 August Complex Fire, which comprised 38 separate fires that were considered a single a massive 418,000 ha fire (Cal Fire 2020 ). Thus, the merging of these multiple fires into a larger event is certainly a factor affecting “fire” size. Indeed, some 2020 fire complexes included multiple fires that never actually merged; for example, the LNU Complex Fire, which ranked within the top 12 fires (Table 3 ), actually comprised several distinctly separate fires that apparently did not merge (San Francisco Chronicle 2020 ).
It has been contended that large fires in the past were often very different in nature from contemporary large fires. For example, many southwestern US mixed conifer forest large fire events in the nineteenth century were low-intensity surface fires, unlike contemporary large fires that are dominated by high-intensity crown fire (Keane et al. 2008 ). This contention, however, varies from descriptions of the top 12 fires recorded here (Table 3 ). For example, when describing the 1889 Plumas fire, newspaper reports state “A large amount of timber and fire wood [were] destroyed.” One report describes the 1891 Eldorado fire as “the most terrible forest fire ever experienced in California…fanned by a strong north wind has swept over almost the entire stretch of country between Georgetown and Salmon Falls…Magnificent forests of a few days ago have been burned over and blackened and lofty pines seared and killed. The scene at night baffles all powers of description, there being a moving mass of fire as far as the eye can reach.” The 1909 Santa Cruz fire was described as “this large conflagration spread… [and] the country is entirely burned over; the entire growth on Loma Prieta Peak and its sides down to Los Gatos Creek is a charred area.”
In general, very few of the large fires reported in (USDA Forest Service 1939-1941 ) were described as low-intensity surface fires. This source described forest fires up and down the state as high intensity conflagrations. For instance, in San Diego County, the 19,000 ha fire of 1870 was described as “the fires which have been raging in the mountains …are wholly unprecedented in extent and …destruction of timber”; in the San Luis Obispo 1869 40,000 ha fire “a great deal of timber and grass has been destroyed”; in Calavaras County in 1889, an 81,000 ha fire was described “A large forest fire has been raging…a large scope of timber country has been laid in waste”; a description of the Tehama 1889 30,000 ha fire was “The forest fire that has raged...was very destructive”, etc. In short, there is little in these records to suggest that nineteenth century large fires were normally less destructive of natural resources than twenty-first century fires. This of course is not meant to negate the commonly accepted paradigm that California forests in the past frequently burned with low-intensity surface fires (Skinner and Chang 1996 ), but that once fires reached epic proportions, and consequently burned through a mosaic of vegetation types, fire behavior appears to have been quite different.
However, one thing that is different between historical large fires and recent ones is that contemporary large fire events are often much more destructive in terms of loss of lives and property. For example, the 2018 Butte County Camp Fire driven by extreme foehn winds killed 85 people and destroyed over 18,000 buildings, however, a similar foehn wind driven fire occurred in Eldorado County in 1891 (Table 3 ), and there were no reports of fatalities and relatively few structures were lost (USFS 1939-1941 ). The difference is due to changes in human demography, e.g., California population throughout the nineteenth century was fewer than 2 million people in contrast to 2020 with a population approaching 40 million. Pressure to find affordable housing has resulted in urban sprawl into watersheds of dangerous fuels (Syphard et al. 2007 , 2019 ). In addition, population growth has played a role in increasing ignitions as most fires that result in human losses are of human origin (Keeley and Syphard 2018 ).
Another factor that is very different in recent decades, when compared to the middle of the century, is the frequency of large fires, with 2007, 2008, 2017, 2018, and 2020 all being peak years for number of large fire events. However, the 1920s were comparable to these recent decades, and in fact, 1929 was a peak year for frequency of large fire events (Fig. 1 b). The1920s decade was also a peak in most regions (Fig. 3 ). Although there was less structure loss in the 1920s, demographic changes could have been involved in terms of frequency of large fires, as the 1920s saw a major influx of people. In this decade, there were increased anthropogenic ignitions driven by greater access to wildlands due to rapid road construction and an order of magnitude increase in car licenses (Keeley and Fotheringham 2001 ).
Climate is widely viewed as a determining factor in fire size, and in particular, drought has been a major driver historically (Little et al. 2016 ; Madadgar et al. 2020 ; Huang et al. 2020 ). One of the important factors behind the 2020 fire events was the anomalously long and intense drought the region experienced beginning in 2012. This drought was experienced across the southern US (Rippey 2015 ) and lasted 3–5 years in California; it was considered to be one of the most severe droughts in California history (Robeson 2015 ; Jacobsen and Pratt 2018 ). The greatest number of recent large fires and size of these fires have been concentrated in the years since this drought (Fig. 4 ). Drought has also been implicated as a factor in other large California fires during the first decade of the twenty-first century (Keeley and Zedler 2009 ) as well as with large fire events in the 1920s, as shown in this study.
While a clear climate signal in terms of drought is a likely driver of big fire events in the state, an emerging issue is the role of anthropogenic climate change (Williams et al. 2019 ). While droughts have historically been a natural occurrence in California’s Mediterranean climate ecosystems, it has been postulated that global warming has made these droughts more severe. Estimates are that the 2012–2014 drought in the Sierra Nevada was perhaps 10–15% more severe due to global warming (Williams et al. 2015 ). This has important implications for the impact of drought on tree and shrub dieback that increases hazardous fuels and contributes to increased fire risk (Stephens et al. 2018 ). However, the relationship between drought and tree dieback in the state is complicated and impacted by competition and other factors (Das et al. 2011 ; Young et al. 2017 ).
The severity of the 2020 fire season in California is not the result of any one factor such as climate change but the result of the “perfect storm” of events. Winter and spring precipitation in the northern part of the state was only about 50% of average, August had a stream of dry lightning storms in northern California that ignited over 5000 fires (Cal Fire 2020 ), there was an intense heat wave in early September that elevated temperatures to record breaking levels (NOAA 2020b ), and forests in the northern half of the state had anomalous fuel loads due to a century of fire suppression and greatly exacerbated by the intense drought of 2012–2015 (Stephens et al. 2018 ).
It is a major challenge to parse out the role of anthropogenic climate change in driving 2020 fires. Certainly, the below normal rainfall year in the north fell within the natural range of variation. The extraordinary lighting storm was perhaps more severe than what is seen in most years, but was not at all unprecedented; e.g., in 2008 northern California experienced a similar event with over 6000 lightning strikes and burning over 400,000 ha from these fires alone, and this is a common phenomenon at a decadal scale, e.g., 1999, 1987, 1977, 1955 (Cal Fire 2008 ). Further contributing to the 2020 fires was the intense heat wave that may be linked to climate change (Gershunov and Guirguis 2012 ; Hully et al. 2020 ). The role of anomalous fuel accumulation due to more than a century of fire suppression and made much worse by 2012–2016 drought was also a major contributor to the size of these fires.
Historically, California fires as big as some of the largest fires in 2020 year have occurred as evident from records beginning in 1860. However, without question, 2020 was an extraordinary year for fires in California. This was driven by a multitude of factors but prominently is the extraordinary droughts the state has experienced in the last couple decades. Peaks in the number of large fires have occurred in the 1920s as well as in the twenty-first century and both occurred in decades with extended droughts.
Availability of data and materials
Data from published sources listed in the “Methods” section.
Abbreviations
California Department of Forestry and Fire Protection
Cal Fire’s Fire and Resource Assessment Program
National Oceanic and Atmospheric Administration
Palmer Drought Severity Index
Abatzoglou, J.T., A.P. Williams, and R. Barbero. 2019. Global emergence of anthropogenic climate change in fire weather indices. Geophysical Research Letters 46 (1): 326–336. https://doi.org/10.1029/2018GL080959 .
Article Google Scholar
Bailey, R.G. 1980. Description of the ecoregions of the United States. Misc. Publication 1931 . Washington, D.C: U.S. Department of Agriculture.
Google Scholar
Baker, W.L. 2014. Historical forest structure and fire in Sierran mixed-conifer forests reconstructed from General Land Office survey data. Ecosphere 5 (7): 79.
Barrett, L.A. 1935. A record of forest and field fires in California from the days of the early explorers to the creation of the forest reserves . San Francisco: USDA Forest Service.
Cal Fire. 2008. June 2008 California Fire Siege Summary Report . Sacramento: Cal Fire.
Cal Fire. 2020. Fire incidents. https://www.fire.ca.gov/incidents/
Cermak, R.W. 2005. Fire in the forest: a history of forest fire control on the national forests in California, 1898-1956 . Washington, D.C.: US Government Printing Office.
Cook, E.R., D.M. Meko, D.W. Stahle, and M.K. Cleaveland. 1999. Drought reconstructions for the continental United States. Journal of Climate 12: 1145–1162 ftp://ftp.ncdc.noaa.gov/pub/data/paleo/drought/NAmericanDroughtAtlas.v2/ .
Das, A.J., J. Battles, N.L. Stephenson, and P.J. van Mantgem. 2011. The contribution of competition to tree mortality in old-growth coniferous forests. Forest Ecology and Management 261 (7): 1203–1213. https://doi.org/10.1016/j.foreco.2010.12.035 .
Diaz, H.F., and T.W. Swetnam. 2013. The wildfires of 1910. Bulletin of the American Meteorological Society 94 (9): 1361–1370. https://doi.org/10.1175/BAMS-D-12-00150.1 .
Gershunov, A., and K. Guirguis. 2012. California heat waves in the present and future. Geophysical Research Letters 39: L18710.
Goforth, B.S., and R.A. Minnich. 2007. Evidence, exaggeration, and error in historical accounts of chaparral wildfires in California. Ecological Applications 17 (3): 779–790. https://doi.org/10.1890/06-0831 .
Article PubMed Google Scholar
Greenlee, J. M. and A. Moldenke. 1982. History of wildland fires in the Gabilan Mountains region of central coastal California. Unpublished report under contract with the National Park Service.
Guttman, N.B., and R.G. Quayle. 1996. A historical perspective of U.S. climate divisions. Bulletin of the American Meteorological Society 77 (2): 293–303. https://doi.org/10.1175/1520-0477/WF13115 .
Howard, L.F., G.D. Cahalan, K. Ehleben, B.A. Muhammad El, H. Halza, and S. DeLeon. 2021. Fire history and dendroecology of Catoctin Mountain, Maryland, USA, with newspaper corroboration. Fire Ecology 17 (1): 8. https://doi.org/10.1186/s42408-021-00096-2 .
Huang, Y., Y. Jin, M.W. Schwartz, and J.H. Thorne. 2020. Intensified burn severity in California’s northern coastal mountains by drier climatic condition. Environmental Research Letters 15 (10): 104033. https://doi.org/10.1088/1748-9326/aba6af .
Hully, G.C., B. Dousset, and B.H. Karn. 2020. Rising trends in heatwave metrics across southern California. Earth’s Future 8: e2020EF001480.
Jacobsen, A.L., and R.B. Pratt. 2018. Extensive drought-associated plant mortality as an agent of type-conversion in chaparral shrublands. New Phytologist 219 (2): 498–504. https://doi.org/10.1111/nph.15186 .
Keane, R.E., J.K. Agee, P. Fule, J.E. Keeley, C. Key, S.G. Kitchen, R. Miller, and L.A. Schulte. 2008. Ecological effects of large fires on US landscapes: benefit or catastrophe? International Journal of Wildland Fire 17 (6): 696–712. https://doi.org/10.1071/WF07148 .
Keeley, J.E., and C.J. Fotheringham. 2001. Historic fire regime in Southern California shrublands. Conservation Biology 15 (6): 1536–1548. https://doi.org/10.1046/j.1523-1739.2001.00097.x .
Keeley, J.E., and A.D. Syphard. 2017. Different historical fire-climate relationships in California. International Journal of Wildland Fire 26 (4): 253–268. https://doi.org/10.1071/WF16102 .
Keeley, J.E., and A.D. Syphard. 2018. Historical patterns of wildfire ignition sources in California ecosystems. International Journal of Wildland Fire 27 (12): 781–799. https://doi.org/10.1071/WF18026 .
Keeley, J.E., and S.D. Syphard. 2019. Twenty-first century California, USA, wildfires: fuel-dominated vs. wind-dominated fires. Fire Ecology 15: 24. https://doi.org/10.1186/s42408-019-00410 .
Keeley, J.E., and P.H. Zedler. 2009. Large, high intensity fire events in southern California shrublands: debunking the fine-grained age-patch model. Ecological Applications 19 (1): 69–94. https://doi.org/10.1890/08-0281.1 .
Little, J.S., D.L. Peterson, K.L. Rilery, Y. Liu, and C.H. Luce. 2016. A review of the relationship between drought and forest fire in the United States. Global Change Biology 22 (7): 2353–2369. https://doi.org/10.1111/GCB.13275 .
Lombardo, K.J., T.W. Swetnam, C.H. Baisan, and M.I. Borchert. 2009. Using bigcone douglas-fir fire scars and tree rings to reconstruct interior chaparral fire history. Fire Ecology 5 (3): 35–56. https://doi.org/10.4996/fireecology.0503035 .
Madadgar, S., M. Sadegh, F. Chiang, E. Ragno, and A. Agha Kouchak. 2020. Quantifying increased fire risk in California in response to different levels of warming and drying. Stochastic Environmental Research and Risk Assessment 34 (12): 2023–2031. https://doi.org/10.1007/s00477-020-01885-y .
Miller, J.D., E.E. Knapp, and C.S. Abbott. 2021. California National Forests fire records 1911- 1924 . Fort Collins: Forest Service Research Data Archive. https://doi.org/10.2737/RDS-2021-0029 .
Book Google Scholar
Mitchell, J.S. 1947. Forest fire statistics: their purpose and use. Fire Control Notes 8 (4): 14–17.
Morford, L. 1984. Wildland Fires: History of Forest Fires in Siskiyou County . Published by the author.
Nagy, R.C., E. Fusco, B. Bradley, J.T. Abatzoglou, and J. Balch. 2018. Human-related ignitions increase the number of large wildfires across U.S. ecoregions. Fire 1 (4). https://doi.org/10.3390/fire1010004 .
NOAA. 2020a. Historical Palmer Drought Indices. https://www.ncdc.noaa.gov/temp-and-precip/drought/historical-palmers/
NOAA. 2020b. Summer 2020 ranked as one of the hottest on record for U.S. August was remarkably hot and destructive. https://www.noaa.gov/news/summer-2020-ranked-as-one-of-hottest-on-record-for-us
Rippey, B.R. 2015. The U.S. drought of 2012. Weather and Climate Extremes 10: 57–64. https://doi.org/10.1016/j.wace.2015.10.004 .
Robeson, S.M. 2015. Revisiting the recent California drought as an extreme value. Geophysical Research Letters 42 (16): 6771–6779. https://doi.org/10.1002/2015GL064593 .
San Francisco Chronicle. 2020. https://www.sfchronicle.com/projects/california-fire-map/2020- 425 lnu-lightning-complex
Skinner, C.N., and C.-R. Chang. 1996. Fire regimes, past and present. Sierra Nevada Ecosystem Project.: Final report to Congress, vol. II. Assessments and scientific basis for management options , 1041–1069. Davis: University of California Centers for Water and Wildland Resources.
Stephens, S.L. 2005. Forest fire causes and extent on United States Forest Service lands. International Journal of Wildland Fire 14 (3): 213–222. https://doi.org/10.1071/WF04006 .
Stephens, S.L., B.M. Collins, C.J. Fettig, M.A. Finney, C.M. Hoffman, E.E. Knapp, M.P. North, H. Safford, and R.B. Wayman. 2018. Drought, tree mortality, and wildfire in forests adapted to frequent fire. BioScience 68 (2): 77–88. https://doi.org/10.1093/biosci/bix146 .
Syphard, A.D., and J.E. Keeley. 2016. Historical reconstructions of California wildfires vary by data source. International Journal of Wildland Fire 25 (12): 1221–1227. https://doi.org/10.1071/WF16050 .
Syphard, A.D., and J.E. Keeley. 2020. Mapping fire regime ecoregions in California. International Journal of Wildland Fire 29 (7): 595–601. https://doi.org/10.1071/WF19136 .
Syphard, A.D., V.C. Radeloff, J.E. Keeley, T.J. Hawbaker, M.K. Clayton, S.I. Stewart, and R.B. Hammer. 2007. Human influence on California fire regimes. Ecological Applications 17 (5): 1388–1402. https://doi.org/10.1890/06-1128.1 .
Syphard, A.D., H. Rustigian-Romsos, M. Mann, E. Conlisk, M.A. Moritz, and D. Ackerly. 2019. The relative influence of climate and housing development on current and projected future fire patterns and structure loss across three California landscapes. Global Environmental Change 56: 41–55. https://doi.org/10.1016/j.gloenvcha.2019.03.007 .
USDA Forest Service. 1939-1941. Bibliography of Early California Forestry. (69 volumes, WPA Projects 70-10896 and 70-12132, ) housed in the reference department of the University of California . Berkeley: BioScience Library.
Vose, R.S., S. Applequist, M. Squires, I. Durre, M.J. Menne, C.N. Williams Jr., C. Frnimore, K. Gleason, and D. Arndt. 2014. Improved historical temperature and precipitation time series for U.S. climate divisions. Journal of Applied Meteroology and Climatology 53 (5): 1232–1251. https://doi.org/10.1175/JAMC-D-13-0248.1 .
Williams, A.P., J.T. Abatzoglou, A. Gershunov, J. Guzman-Morales, D.A. Bishop, J.D. Balch, and D.P. Lettenmaier. 2019. Observed impacts of anthropogenic climate change on wildfire in California. Earth’s Future 7 (8): 892–910. https://doi.org/10.1029/2019EF001210 .
Williams, A.P., R. Seager, J.T. Abatzoglou, B.I. Cook, J.E. Smardon, and E.R. Cook. 2015. Contribution of anthropogenic warming to California drought during 2012-2014. Geophysical Research Letters 42 (16): 6819–6828. https://doi.org/10.1002/2015GL064924 .
Young, D.J.N., J.T. Stevens, J.M. Earles, J. Moore, A. Ellis, A.L. Jirka, and A.M. Latimer. 2017. Long-term climate and competition explain forest mortality patterns under drought. Ecology Letters 20 (1): 78–86. https://doi.org/10.1111/ele.12711 .
Download references
Acknowledgements
Any use of trade, product, or firm names is for descriptive purposes only and does not imply endorsement by the US government. We appreciate the comments on an earlier draft of this manuscript by Keith Lombardo.
Support from institutional funds.
Author information
Authors and affiliations.
U.S. Geological Survey, Western Ecological Research Center, Sequoia-Kings Canyon Field Station, 47050 General’s Highway, Three Rivers, CA, 93271, USA
Jon E. Keeley
Department of Ecology and Evolutionary Biology, University of California, 612 Charles E. Young Drive, South Los Angeles, Los Angeles, CA, 90095-7246, USA
Vertus Wildfire Insurance Services LLC, 600 California St, San Francisco, CA, 94108, USA
Alexandra D. Syphard
You can also search for this author in PubMed Google Scholar
Contributions
Data collection and presentation was by the first author: both authors contributed to ideas and writing. The authors read and approved the final manuscript.
Corresponding author
Correspondence to Jon E. Keeley .
Ethics declarations
Ethics approval and consent to participate.
Not applicable.
Consent for publication
Not applicable
Competing interests
The authors declare that they have no competing interests.
Additional information
Publisher’s note.
Springer Nature remains neutral with regard to jurisdictional claims in published maps and institutional affiliations.
Rights and permissions
Open Access This article is licensed under a Creative Commons Attribution 4.0 International License, which permits use, sharing, adaptation, distribution and reproduction in any medium or format, as long as you give appropriate credit to the original author(s) and the source, provide a link to the Creative Commons licence, and indicate if changes were made. The images or other third party material in this article are included in the article's Creative Commons licence, unless indicated otherwise in a credit line to the material. If material is not included in the article's Creative Commons licence and your intended use is not permitted by statutory regulation or exceeds the permitted use, you will need to obtain permission directly from the copyright holder. To view a copy of this licence, visit http://creativecommons.org/licenses/by/4.0/ .
Reprints and permissions
About this article
Cite this article.
Keeley, J.E., Syphard, A.D. Large California wildfires: 2020 fires in historical context. fire ecol 17 , 22 (2021). https://doi.org/10.1186/s42408-021-00110-7
Download citation
Received : 24 April 2021
Accepted : 12 July 2021
Published : 25 August 2021
DOI : https://doi.org/10.1186/s42408-021-00110-7
Share this article
Anyone you share the following link with will be able to read this content:
Sorry, a shareable link is not currently available for this article.
Provided by the Springer Nature SharedIt content-sharing initiative
- fire severity
- historical fires

An official website of the United States government
Here’s how you know
Official websites use .gov A .gov website belongs to an official government organization in the United States.
Secure .gov websites use HTTPS A lock ( Lock A locked padlock ) or https:// means you’ve safely connected to the .gov website. Share sensitive information only on official, secure websites.
https://www.nist.gov/news-events/news/2023/08/nist-issues-new-guidance-emergency-response-during-wildfires
NIST Issues New Guidance for Emergency Response During Wildfires
Lessons learned from the 2018 camp fire in california include strategies for protecting lives when there is not enough time to safely evacuate all residents..
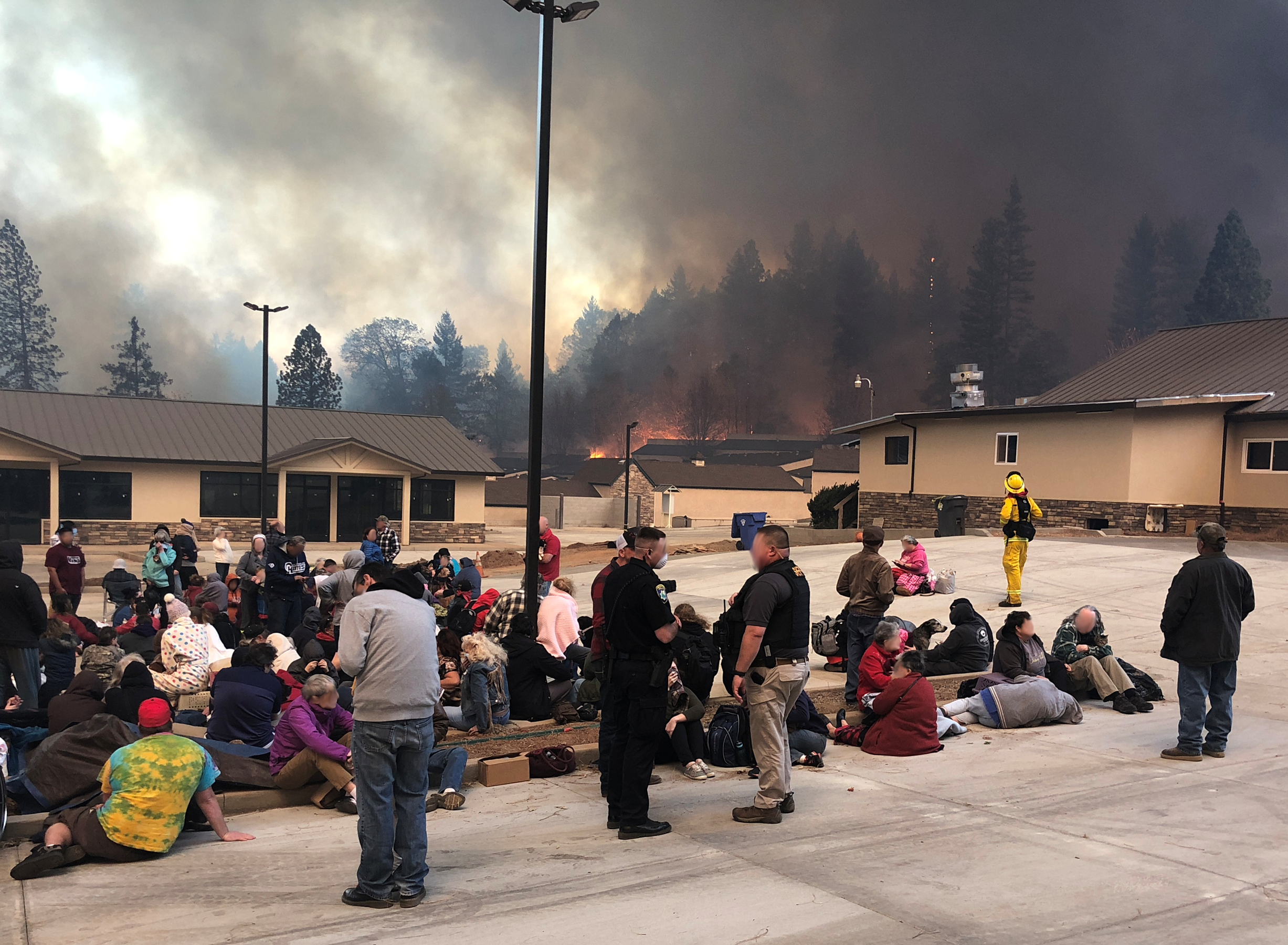
The National Institute of Standards and Technology (NIST) has released a pair of reports that provide guidance for strengthening wildfire preparedness across the United States, including specific actions that communities can take to save lives when there is not enough time to safely evacuate all residents. NIST has also created a new website with guidance for making built structures and entire communities more fire resistant.
The reports are based on an analysis of the Camp Fire, which killed 85 people in California in 2018, and are the culmination of several years of research by experts at NIST and other federal and state agencies.
“The goal of these reports is to apply lessons learned from the Camp Fire in ways that make our communities safer in the face of continuing wildfire risks,” said NIST fire protection engineer Alex Maranghides, an author on the two reports. “Tragically, we are releasing these reports in the aftermath of the fire on Maui Island in Hawaiʻi. Our thoughts are with everyone affected by this terrible event.”
The Camp Fire Case Study Report
The Camp Fire began in Northern California’s Butte County on the morning of Nov. 8, 2018. It spread quickly through the communities of Pulga and Concow and slammed into the town of Paradise a couple of hours later.
More than 40,000 people attempted to evacuate, but they had little time to escape. Heavy traffic combined with deteriorating conditions to produce widespread gridlock. Hundreds of residents experienced a dangerous situation known as burnover, in which fire moved through their locations and exposed them to hazardous conditions. All of this took place in a region that had done advance planning for wildfires.
“The Camp Fire showed us what can happen to a prepared community when there is not sufficient time to evacuate,” said Maranghides.
Shortly after the fire subsided, NIST worked with the California Department of Forestry and Fire Protection (CAL FIRE), the Federal Emergency Management Agency’s U.S. Fire Administration, and the town of Paradise to collect data about the disaster. NIST experts then used that data to build an in-depth case study whose lessons could improve preparedness for wildfires everywhere.
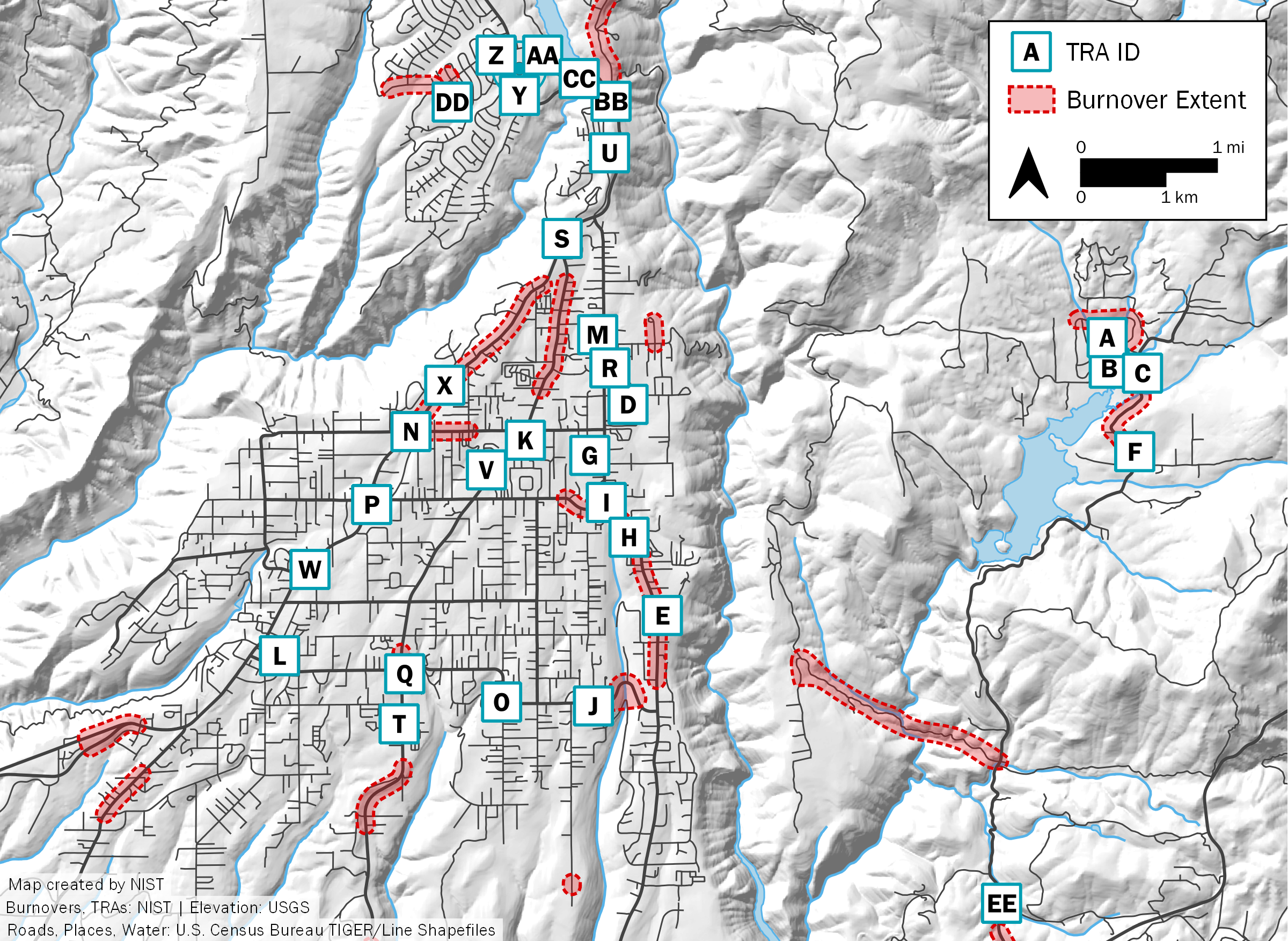
“Evacuations are critical, and seconds count when wildfire threatens. Early and timely notification to the public is critical for survival,” said Steven Hawks of CAL FIRE, a co-author of the case study. “It is important to learn from what occurred during the Camp Fire and use this knowledge to ensure communities are more prepared in the future.”
A new report, A Case Study of the Camp Fire: Notification, Evacuation, Traffic, and Temporary Refuge Areas , meshes 2,600 observations and data points with the timeline of the spread of the Camp Fire to examine how first responders performed lifesaving actions during the historic blaze.
“The coordinated effort among firefighters, law enforcement and other first responders saved thousands of lives. Their actions are especially highlighted by 198 specific rescue events that we were able to document,” said NIST researcher Eric Link, a co-author of the study.
The losses could have been exponentially greater if not for the actions of emergency personnel. In addition to clearing roads and rescuing civilians from buildings, first responders quickly created 31 outdoor shelters known as temporary refuge areas that accommodated more than 1,200 people.
The ESCAPE Report
In addition, NIST researchers and their colleagues distilled the lessons from the Camp Fire into a second report that provides general guidance on wildfire response and makes it easier for communities to apply the research findings. The WUI Fire Evacuation and Sheltering Considerations: Assessment, Planning, and Execution (ESCAPE) report helps communities develop advance plans and process new information during wildfires, all while considering a community’s resources and conditions.
Based on both the pitfalls and successes of Paradise’s emergency response, the authors of the ESCAPE report suggest that evacuation is not a universal solution. Under conditions where there is not time for everyone to flee, it may be better for residents to shelter in their community at a designated safety zone. Ideally, these shelters would be systematically placed and maintained beforehand, so that they are safe and easy to access throughout a community, Maranghides said.
ESCAPE recommends that city officials establish several metrics to gain a comprehensive understanding of their community’s emergency response capabilities. One of the most important metrics is the time it takes to get everyone out of town, a number that can be estimated using evacuation drills and traffic models.
Clocking evacuation times in advance will allow officials to make better decisions in emergency situations. If time permits, then a full evacuation might be in order. If not, then citizens may be directed to their nearest safety zones. The guidance also emphasizes the need for advance planning for the safe evacuation of critical care facilities and people with limited mobility.
“State and local fire service leaders and emergency managers need evidence-based guidance to make informed decisions and guide safe evacuations,” said Nicole LaRosa, who as senior policy adviser to the U.S fire administrator on WUI policies collaborated with NIST on the report. “This report applies research findings to on-the-ground practices that can save lives.”
The Hazard Mitigation Methodology Website
NIST has launched a new website intended to help community leaders and first responders in wildfire-prone areas make buildings and other structures more resistant to fire. The website is based on the Hazard Mitigation Methodology (HMM), which was developed by researchers at NIST, CAL FIRE and other agencies and organizations. While traditional strategies focus on hardening individual structures, the HMM takes a community-wide approach to addressing wildfire risks.
The new website, developed with input from the Consumer Product Safety Commission, includes an overview of the new methodology and its benefits, a guide to implementing the methodology, and drawings and plans that contractors can use to upgrade buildings and multibuilding parcels. The website also includes a list of federal and state grants available to assist communities in implementing the new methodology.
Together, the two reports and the website give communities important new tools for implementing proactive approaches to managing wildfire risks.
“There have been no resources like this for small and medium-sized communities in wildfire-prone areas until now,” Maranghides said. “Many communities don't have the tools they need to plan for large wildfires, but the ESCAPE and the HMM website provide new paths forward.”
Alexander Maranghides et al. A Case Study of the Camp Fire: Notification, Evacuation, Traffic, and Temporary Refuge Areas (NETTRA). NIST Technical Note 2252. July 2023. DOI: 10.6028/NIST.TN.2252
Alexander Maranghides and Eric Link. WUI Fire Evacuation and Sheltering Considerations: Assessment, Planning, and Execution (ESCAPE). NIST Technical Note 2262. August 2023. DOI: 10.6028/NIST.TN.2262
- 281.412.7766
- [email protected]
- Learner Dashboard
- GET YOUR CAUSE MAPPING TEMPLATE

- About Cause Mapping®
- What is Root Cause Analysis?
- Cause Mapping® Method
- Cause Mapping® FAQs
- Why ThinkReliability?
- Online Workshops
- On-Demand Training Catalog
- On-Demand Training Subscription
- Company Case Study
- Upcoming Webinars
- Webinar Archives
- Public Workshops
- Private Workshops
- Cause Mapping Certified Facilitator Program
- Our Services
- Facilitation, Consulting, and Coaching
- Root Cause Analysis Program Development
- Work Process Reliability™
- Cause Mapping® Template
- Root Cause Analysis Examples
- Video Library
- Articles and Downloads
- About ThinkReliability
- Client List
- Testimonials
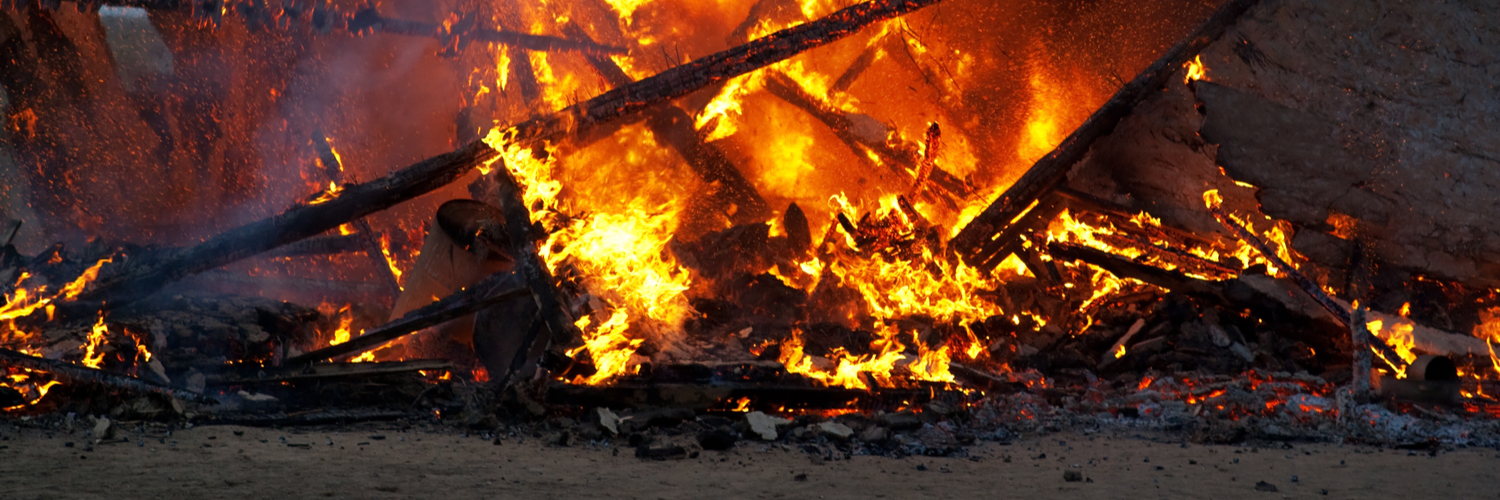
Case Study: The Camp Fire - California's Most Destructive Wildfire
The most destructive wildfire ever.
The Camp Fire, California’s most destructive wildfire in history, burned in Butte County from November 8 through November 25, 2018, claiming at least 86 lives and causing an estimated $16.5 billion in damage. It burned more than 18,000 buildings, including the majority of the town of Paradise.
The “roots” of the problem
With a tragedy of this scope, the natural response is to want to determine why this happened and to figure out how to prevent a similar incident in the future. A Cause Map, a visual format for performing root cause analysis , can be built to dig into the numerous causes that led to this tragic fire. Creating a Cause Map helps organize information and make cause-and-effect relationships clear. The investigation into the fire is still underway, but an initial Cause Map can be developed to document what is known and then updated as more information becomes available.
Like most instances, there is more than one cause that contributed to this incident, and all the causes need to be understood to develop effective solutions that can be implemented to reduce the risk of future catastrophic fires. To view an intermediate level Cause Map of this root cause analysis example, click on the thumbnail below.
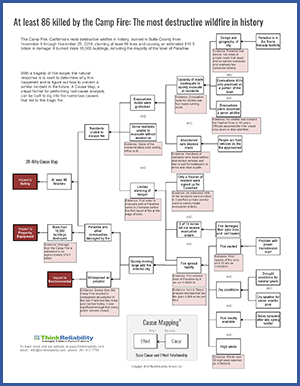
The fire starts
Investigators are still working to determine exactly what started the Camp Fire, but it has been reported in the media that the local electric company detected a problem on a transmission line 15 minutes before the first report of the blaze. Lawsuits allege that a faulty steel ring on a transmission tower resulted in live wires falling and igniting the fire.
The fire quickly grows
While the debate over how the fire started is ongoing, one thing everyone can agree on is that the Camp Fire spread rapidly. Drought over several years and dry weather in the months prior to this fire led to conditions prime for a fire to grow. Grass that had sprouted after a late spring rain dried out over the summer, providing a plentiful fuel source. Mix in high winds and the small fire quickly turned into a raging ember storm.
Unable to evacuate
The Cause Map of this tragedy illustrates that the size and speed of the fire wasn’t the only cause that contributed to the problem. The design and layout of the city of Paradise is also an important factor to consider. Paradise is located in the Sierra Nevada foothills and there are almost 100 miles of private roads that dead-end on narrow overlooks with relatively few streets connecting them. The evacuation route out of Paradise for 38,000 people was four roads running south. Evacuations routes were quickly gridlocked as residents attempted to flee to safety from the approaching fire.
Emergency planning
In preparation for a wildfire scenario, community leaders in Paradise had emergency plans and practiced evacuation drills, but the plans assumed an approaching fire would be a tamer wildfire like what the city had experienced in the past. There was no plan for how to evacuate the entire city at one time. The roads out of Paradise were gridlocked within an hour of the first evacuation notice . Three hours later, hundreds of people were trapped by flames within the city.
Additionally, the public alert system was problematic. Not all residents were signed up for CodeRed, a service that provided evacuation orders for the city. Communications were also compromised as fiber optic lines and cell towers were damaged by fire. Many of the residents never received an evacuation order.
Plan for the worst-case emergency
The lessons learned from this root cause analysis example go far beyond city planning. Every organization needs to develop a plan for how to respond to an emergency or natural disaster. Emergency plans are essential for a quick and effective response when a disaster strikes. Planning for common emergencies is useful, but planning for worst-case scenarios is also necessary because sometimes they become reality.
What's Next?
Ready to learn how to break down your own problems? Our 5-Why Online Short Course teaches people to apply cause-and-effect thinking to break down daily issues. This course is for frontline problem solvers, technical leads and management. It’s applicable to safety, environmental, operations, maintenance and administrative functions.
Share This Post With A Friend

Similar Posts
Other resources.
- Root Cause Analysis blog archive
- Patient Safety blog archive
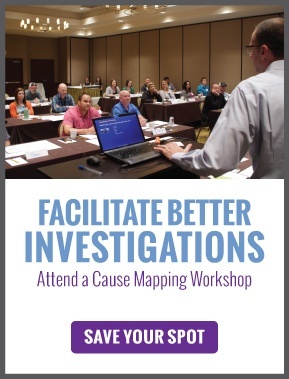
READ BY - - - - - - - - - -

Other Resources - - - - - - - - - -

Sign Up For Our eNewsletter
Language selection
- Français fr
Canada’s record-breaking wildfires in 2023: A fiery wake-up call
In 2023, Canada is facing a formidable challenge: record-breaking wildfires. These fires are fuelled by record high temperatures and widespread drought conditions across the country. To what extent can climate change be linked to these events? Scientists from around the world did a rapid turn-around study to find answers.
The 2023 experience:
Canada’s 2023 wildfire season is the most destructive ever recorded, and it’s not over yet. By September 5, more than 6,132 fires had torched a staggering 16.5 million hectares of land. To put that in perspective, that’s an area larger than Greece and more than double the 1989 record. Normally, an average of 2.5 million hectares of land are consumed in Canada every year. And unlike previous years, the fires this year were widespread, from the West Coast to the Atlantic provinces, and the North. By mid-July, there were 29 mega-fires, each exceeding 100,000 hectares.
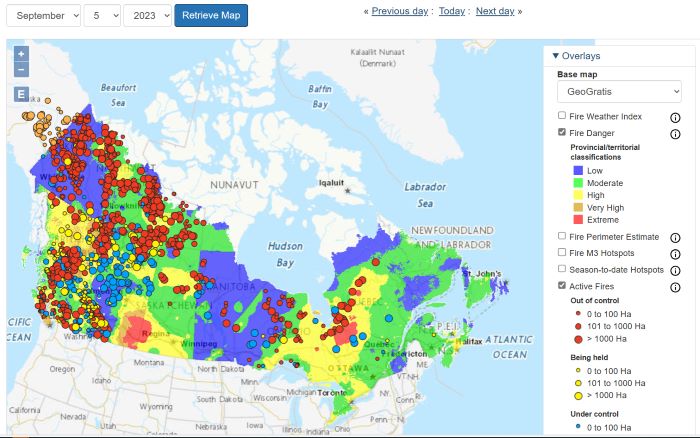
Screen grab from NRCan’s interactive map showing active fires as of September 5, 2023.
“The word ‘unprecedented’ doesn’t do justice to the severity of the wildfires in Canada this year,” says Yan Boulanger, research scientist in forest ecology at Natural Resources Canada. “From a scientific perspective, the doubling of the previous burned area record is shocking.”
Learning from this year’s fires
Yan and two other Canadian Forest Services scientists — Jonathan Boucher and Sandy Erni — were part of an international team of 16 scientists involved in a rapid research study focused on Quebec’s wildfire season. The team found that climate change more than doubled the likelihood of extreme fire weather conditions in Quebec.
The study was conducted by World Weather Attribution (WWA), a group that looks at how climate change affects the intensity and likelihood of an extreme weather event. These studies are done very quickly, and while it’s important to note that this report hasn’t been peer reviewed yet, the study used peer-reviewed methods.
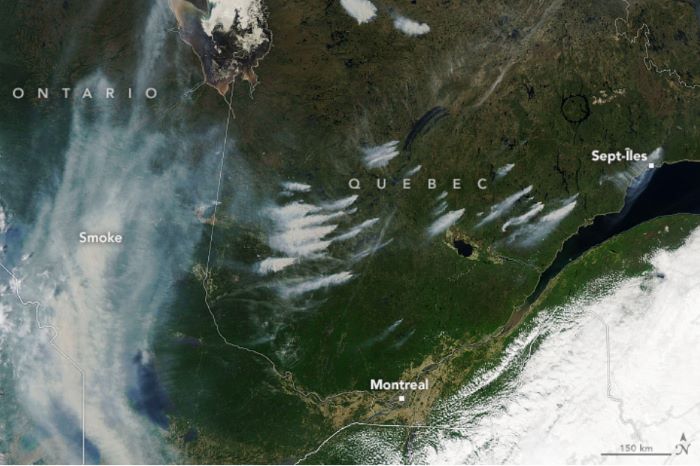
June 3, 2023 — The Moderate Resolution Imaging Spectroradiometer (MODIS) on NASA’s Aqua satellite captured this view of smoke billowing from Quebec fires on June 3. On June 1 more than 120 fires were ignited in a single day, and as of June 5, more than 150 wildfires were active in Quebec. (NASA Earth Observatory image of the Quebec wildfires by Lauren Dauphin, using MODIS data from NASA EOSDIS LANCE and GIBS/Worldview)
Why study Quebec? Well, Canada is so big that it’s difficult to gather data from across all time zones to capture data across the country from coast to coast in a timely manner. Also, Quebec had an early start to the devastating 2023 wildfire season. Warm and dry conditions appeared in early May, setting the stage for June and July. On June 1, more than 120 fires were ignited by lightning in just one day. “Climate change is greatly increasing the flammability of the fuel available for wildfires because the trees, fallen trees, and underbrush are all so dry,” explains Yan. “This means that a single spark, regardless of its source, can rapidly turn into a blazing inferno.”
From June 1 to 25, more land burned in southern Quebec than in the previous 20 years combined. These conditions led to the largest single fire ever recorded in southern Quebec, which consumed 460,000 hectares. With all this, it’s no wonder scientists are trying to find out what’s going on.
Researchers used Natural Resources Canada’s Fire Weather Index (FWI), a metric that combines temperature, windspeed, relative humidity and precipitation to estimate the level of fire danger. To assess the effect of hot and dry weather conditions from January to July, the scientists also calculated the Cumulative Daily Severity Rating from the FWI.
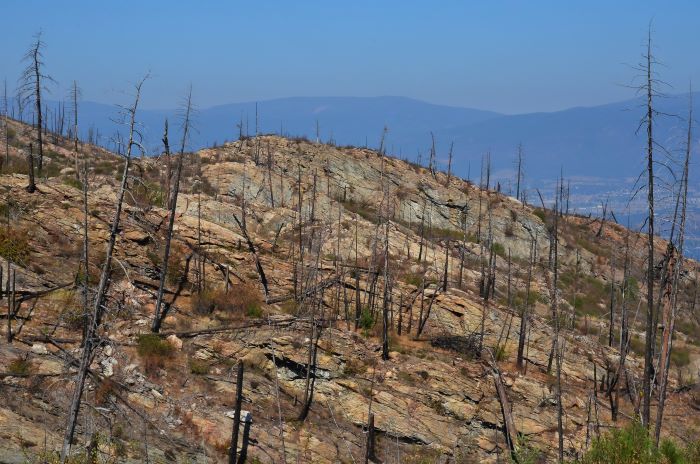
Aftermath of a wildfire near Kelowna, B.C. This year’s fire activity could also impact forest carbon balance, biodiversity, and disrupt local businesses, forest sector economies and Indigenous communities. (photo: Getty Images)
The role of climate change
“There’s no question, extreme weather, record high temperatures and dry conditions caused by climate change intensified this year's wildfire crisis,” says Jonathan.
Canada experienced its warmest May to July period in over 80 years, breaking previous national temperature records for the two-month period by 0.8°C. To quantify the effect of climate change on weather, scientists used weather data and computer model simulations to compare the pre-industrial climate to today’s conditions, after about 1.2°C of global warming since the late 1800s. The study found that climate change made the extreme intensity of this fire season at least two times more likely than under preindustrial climate while the persistence of these conditions were at least seven times more likely. Furthermore, they found that wildfire-prone weather conditions in Quebec were 50 percent more intense because of climate change caused by human activity, while the peak intensity recorded was 20 percent higher.
Assessing events such as wildfires and weather presents a unique challenge. Jonathan emphasizes the need to constantly update climate models to account for ever-evolving weather patterns. “For instance, this summer’s record temperatures and low humidity in Quebec was extreme by today’s standards,” he says. “But they could be the norm by the end of the century.”
Long-term consequences :
The increasing risk of wildfires highlighted by the WWA study raises some key questions: How do we prepare? How do we adapt? What about wildfire response, containment and recovery? The WWA publishes results soon after the event, instead of waiting for a long review process, so they can provide context and lessons learned quickly after an extreme event.
This year’s fire activity could also impact forest carbon balance, biodiversity, and disrupt local businesses, forest sector economies and Indigenous communities.
Looking ahead:
As wildfire risks will continue to intensify as the climate warms, Canada needs to reduce fossil fuel consumption and adapt fire management and mitigation strategies. There are different ways to manage wildfire risk, including controlled burns, revised forestry guidelines, and procedures to manage fuel around communities and infrastructure. It’s a balance of these techniques that can help deal with future challenges.
“A changing climate means a proactive approach must be taken,” says Sandy Erni. “Studies like this can offer important lessons to be better prepared for the next time a devastating event like this occurs.”
For more information:
Canadian Wildland Fire Information System
Fire Weather Maps (Natural Resources Canada)
World Weather Attribution
Impact of climate change on forest fires (Simply Science podcast)
Related stories
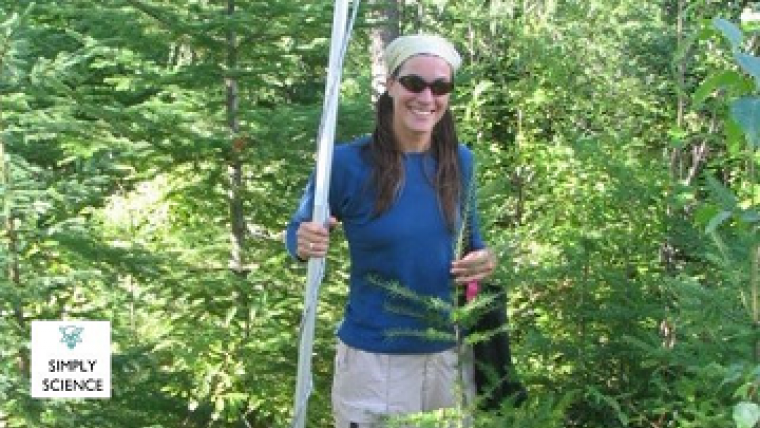
Marie-Claude Gros-Louis, who works at the Laurentian Forestry Centre (LFC) of the Canadian Forest Service, is a professional appreciated by both her colleagues and her clients. She is a triathlete and an entrepreneur, and her generosity toward the causes that are dear to her seems to have no limits!
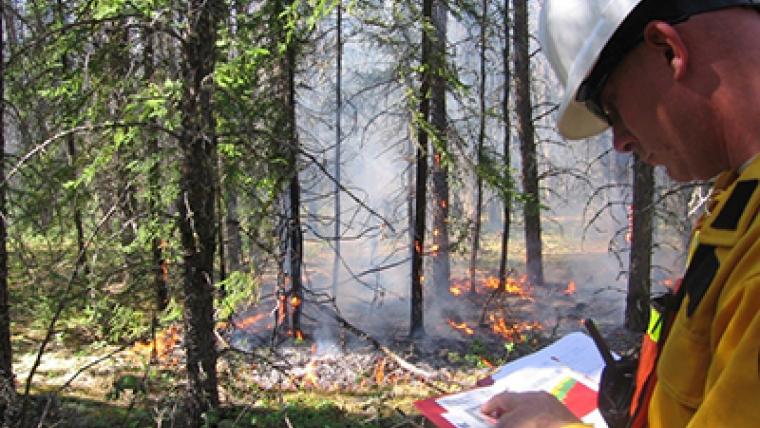
With all of the tools, supplies and gear that fire managers need, the last thing you would expect them to take to a wildfire is a book.
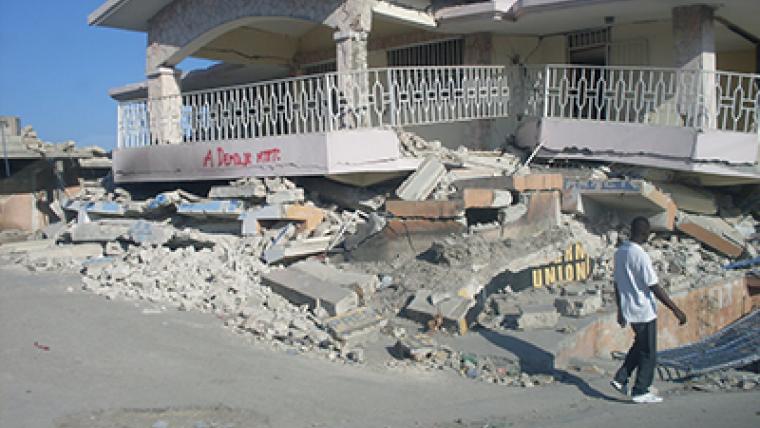
Media reports of earthquakes often identify their epicentre as a single point on a map. This may be accurate for small earthquakes. But for large ones, the seismic energy is released from a large surface, not a point on a map.
Simply Science
- Search Simply Science
- Resource Packets
- Media Relations
- Sign up for our newsletter
Stay connected and follow us on any of our social media channels
Page details
- Case Studies
- Teaching Guide
- Using the Open Case Studies Website
- Using the UBC Wiki
- Open Educational Resources
- Case Implementation
- Get Involved
- Process Documentation
Fort McMurray and the Fires of Climate Change
Fort McMurray, located in a beautiful service area with vast oil sands deposit in northeast of Alberta, is surrounded by peatlands and boreal forests of aspen and spruce. Though normally very wet, Fort McMurray is relative dry and hot in summer, and its weather is becoming more extreme because of climate change. With a low moisture content and a high volume of resin for defence against disease, stems of trees in boreal forests are highly flammable. Beginning southwest of Fort McMurray, Alberta on May 1st, 2016, the wildfire swept through forests and communities and destroyed around 2,400 buildings in only two days, resulting in over 8,000 residents to leave their homes. Ultimately, it was under control by July 5th, 2016. The fire, reported spreading cross 590,000 hectares, is the costliest disaster in Canadian history. The intense forest fire has a profound impact on local environment and ecology. About 500 species of wildlife lost their habitats or were in danger due to the fire. Many wastes such as ash and rotting food were produced in the burning process. The fire also released a large amount of CO2 and other toxic contaminants including heavy metals and PAHs deposited on trees and soils.
On May 1st, 2016 at 9:57pm (MDT), a wildfire began to sweep the Centennial Trailer Park and the neighborhoods of Prairie Creek and Gregoire at the southwest of Fort McMurray, Alberta, Canada. On May 3rd, 2016 [1] , this event was described as the largest wildfire event in Alberta’s history. Canadian military, Royal Canadian Mounted Police, Canadian provincial agencies, 300 South African firefighters and many charitable organizations, such as the Canadian Red Cross, all participated into the rescue work in this fire event. Numerous rescue teams came to help the residents in McMurray to evacuate from the extremely unsafe communities. The Canadian Red Cross provided McMurray residents with many medical treatment and donations [1] .
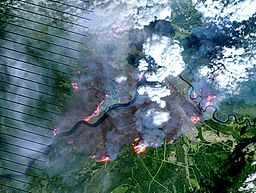
Fort McMurray is a beautiful urban area which covers 59.89 km² in the northeast of Alberta, Canada. This city is surrounded by boreal forest and 2 main rivers: Athabasca river and Clear Water river. There are also several peat bogs within the forest. The whole Boreal forest is located in a flat plain with few hills. And the Rocky Mountains are on the west side of this plaint. The forest fire was started at the southwest of the urban service, and expanded around especially the southeast side.
The wildfire lasted for two months in Fort McMurray, Alberta, Canada, and it started to be under control by July 5th, 2016. The wildfire spread cross 590,000 hectares (1500,000 acres) from the edge of the southwest of Fort McMurray to the northern Alberta, and finally stopped in Saskatchewan, a province with prairie and boreal that bordering the west of Alberta. [3] The sweeping of the wildfire destroyed approximately 2,400 houses and buildings in the southwest of Fort McMurray. Nearly 8,000 residents had to move out of their quarters due to the severe contamination. However, the fire was not under control after sweeping in southwest of Fort McMurray. It became severer and began to spread across the northern part of Alberta and Saskatchewan [2] . Thousands hectares of forest area and a huge number of Athabasca oil sands, one of the three major oil sands deposits of bitumen and extremely heavy crude oil, in Alberta, were further destroyed with the expanding of wildfire By July 5th , 2016, only some small areas were contaminated by the McMurray wildfire and it was reported to be under controlled. Although the fierce spreading of the wildfire was controlled in early July, smoldering fire still continued in Fort McMurray, Alberta. The smoldering fire was not stopped to give negative impacts on natural resources and human beings until the spring of 2017
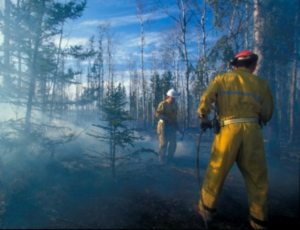
Wildfires in Canada’s forests are very common. Ecological professionals and fire management departments often see fire as a beneficial thing for maintaining the ecological values of forests [3] . According to the statistics in 2016, fires in Canada’s boreal forest burn an average of 2 million hectares of forest every year [3] . The large McMurray wildfire spreading, however, had enormous negative impacts on local environment, ecology, economic activities and society. How can the McMurray burning be special on the Canada’s wildfire history?
Negative impacts on local environmental and ecology
The explosive burning brought a lot of air pollution to Fort McMurray. The large amount of CO 2 released by the burning increased the “greenhouse” effect in Fort McMurray area [4] . The burning of cars, factories and buildings brought hundreds of mercury, many other heavy metals and polycyclic aromatic hydrocarbons (PAHs), which fell on the tree branches and soil [4] . Because contaminants made the air toxic, humans and animals had to breath thousands of small toxic particles into their lungs. As a result, air pollution leads to a huge negative impact on human and animals’ health. In addition to the air pollution, the burning in Fort McMurray also contaminated water [4] . Mercury, polycyclic aromatic hydrocarbons (PAHs) ,and other heavy metals would not only fell onto the forest floors, but also into rivers and streambanks [4] . Athabasca River was the one that received most severe contaminations [5] . Athabasca River became a spot fire during this event [5] . Rainwater in McMurray settled down toxins into the rivers and streambanks. Water previously used by people and animals nearby became toxic during the explosive burning period.
Forests damages and Habitat losses
Forests in Fort McMurray area are mainly mixed boreal forests [4] . The recent dry climate is the main cause of the severity of this explosive wildfire. Tree roots and soils were scorched. The tree species composition and forest type would be changed by this event. Due to the burning in forests areas, about 500 species lost their habitats and now living in danger due to the fire contamination [4] .
Many wastes such as ash and rotting food were produced in the burning process [4] . Waste in the natural areas like forests would not greatly influence people’s daily life in Fort McMurray area. However, it became a serious issue when it continually accumulated in the crowded communities where McMurray people living. Two major contributions of the wastes are toxic ashes and rotting food [4] . Debris and fire-damaged concretes from vehicles, furniture, and public infrastructure including sidewalks and benches in parks were stacked on streets and destroyed constructions after the fire [4] . Communities that people used to happily live in became ruins after the explosive burning. A lot of works needed carrying out to clean the wastes in Fort McMurray area.
Negative impacts on economic and social activities
As people known, the development of oil sand industry in Alberta has promoted the economy of Alberta and Canada over decades [6] . The oil sand production industry in Alberta created hundreds of employment opportunities and contributed millions of tax revenue for the local and federal government. In the reports of assessment of the Fort McMurray wildfire, released by the mid-May in 2016 in Ottawa, two major negative economic impacts are described in the following:
- 1 The Fort McMurray wildfire drops the overall GDP of Alberta to a negative value in the year of 2016. Its economic impact on the national overall GDP is not noticeable.
- 2 Shutting down of the oil sand production actually slowed or even stopped the major economic activity of the oil production industry in Alberta. According to the statistics in the Report of Economic Impacts of the Fort McMurray Wildfires, 1.2 million barrels’ oil were lost per day on average for 14 days. This huge amount of oil lost was translated to direct money lost, equaling roughly $985 million real GDP and 0.33 per cent out of Alberta’s GDP in 2016 [7] .
Layering Perspectives: Other Costs and Impacts?
The information above outlines some of the impacts associated with wildfire in Fort McMuray from the perspective of a natural resources conservation student. In this section, we welcome contributions from other perspectives. Those interested in contributing to this case study may use the following questions as a guide:
- What other costs and/or impacts become apparent when wildfire is viewed through the lens of other disciplines and professions?
- What special expertise, resources, or theoretical orientations might others bring to help us better conceptualize the costs and/or impacts associated with wildfire?
However, there is always another loss that cannot be calculated by these statistics. That is the influence on people’s lives and livelihoods [7] . Over 8,000 residents moved away from their homes and lost their personal property during Fort McMurray wildfires. One thing that is worth being happy during this disaster is that the depressions mood among stricken people in Fort McMurray did not bring a lot of negative impacts on economic and social activities. A rebuilding fund was added to the real GDP in Alberta’s economy in 2017, and it contributed to roughly 0.4% of the overall GDP increase of Alberta. Rebuilding of the lost assets promoted more economic and social activities than before [7] . People in McMurray returned to their homes after the disaster in the early spring of 2017. Funds for rebuilding compensate the lost capitals, and provide many employment positions for the returned residents. However, there is no doubt that the Fort McMurray Wildfires is one of the costliest natural disasters in the history of Alberta. In short, what makes this wildfire special and costly is its intensity, severity and location, which is in the central part of the oil sand industry in Alberta.
Analyzing the fire from local climate and ecosystem
Fort McMurray is located in the Boreal Plains Ecozone, where 84% of the land is covered by forest and less than 20% of the land is used by agriculture. [8] Spruce, pine and aspen are the most common species in this area. These conifer trees have high concentration of resin, which are susceptible to fire. During the long time evolution, these species and the ecosystem have adapted to wildfire. According to the climate record from McMurray airport, the summer starts from May with the average temperature of 9.8°C calculated by the data between 1981and 2010. The highest temperature is around 17°C and the mean precipitation is 36.5mm (33.5mm in rainfall and 3.5mm in snowfall each). June and July have the highest temperatures in record with the precipitation increasing dramatically to 73.3mm and 80.7mm separately. [9]
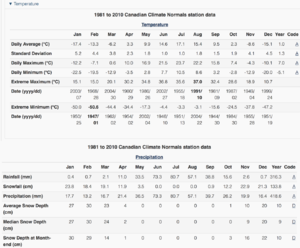
However, the max temperature in 1st May in last year was 27.3°C, which is much higher than the historic records. The foresters in Fort McMurray said the trees in 2015 have suffered a summer drought. And this trend was kept to 2016, caused by the high temperature with low precipitation in 2016. The climate change was considered to be the direct effect to this disturbance. [10] According to the Fir Behavior Triangle, this large fire is not that hard to understand. By analysing the data above, we can easily find the summer in McMurray are used to being pretty warm and wet. Water storage in the whole range of forest is enough for tree species to live during the growing season. The roots of trees living in this area are quite shallow, since they do not have to extend very deep to gather water. Tangled and twisted roots living in the shallow soil with a lot of air is easy to be burned but hard to be put out during the burning. As a result, expanded fire were out of control even if firemen cut several trees to create a gap to separate the fire and unburned forests because of underground burning. [10] By the time goes, precipitation did helped a little bit during the extinguishing of the fire when summer came in June and July. [11]
Analyzing the fire from local economic structure
Fort McMurray is famous for its Athabasca oil sand which is the largest oil sands deposit in the world (143×10 9 m 3 in total). [12] These large amount of oil sands is another fuels in this huge fire. What is more, the file expanded faster than we expected because of these oil sand. Even there is nothing to burn above the ground, the fire can still expand below the ground by soil sands. Even precipitation or crown cutting can hardly stop these underground fire.
Since the oil sands are stored below the ground surface, the most useful way to get the oil sands is digging them and extracting while the precondition of using this method is clear cutting all the plants above the ground. As a result, large are of forest were damaged by oil sands industry until 2009 when the new technology allowed extracting oil sands without disturbing the species. But the new technology is much more expensive and with low extract rate, digging the oil sands is still the most popular way in oil companies today. [13] Losing the forest in this area ruined the local environment and climate a lot. The water storage ability has dropped down a lot because of losing plants. Usually, the roots of plants cooperate with the soil system to maintain the water balance for all the species in this area. Summer drought is going to be a serious problem to all the species left in this area. Furthermore, photosynthesis can reduce the CO2 to reduce the climate change. Clear cutting speed up the climate change to create a more horrible climate for forest to live. "Climate change models and research all point to the idea that fire season is going to be longer in the coming years, and the fires will be more severe," professor David Andison in the faculty of forestry at UBC said. [14]
Energy development is the pillar industry in Alberta which is the largest contribute to the GDP and investments in the states. [15] Moreover, people in Alberta believe that they have the potential to be the the leading energy provider in the world. [16] According to the job survey investigated by the Government of Alberta, 51% of the labor force in Fort McMurray were hired by oil and gas industry directly and most of other people are doing the relative works of energy industry in 2005. [17] It’s not hard to imagine how huge effects this fire caused to Fort McMurray. People not only lost their home, but also lost their jobs. The local economy could hardly be built up in a short time because of their high reliability to the energy industry. Since Athabasca is the largest oil sand deposit in the world, the oil price was influenced by this wild fire last year. [18]
Options for emergency operations and Recommendations
When the wildfire happened on May 1st 2016, the government implemented the emergency operations rapidly [19] . On May 3nd, a large evacuation was addressed by the government, leading to disorder to some degree unavoidably [19] . However, considering of the safety of all residents in Fort McMurray area, it is still a rational and timely act [19] . The Provincial Operations Centre (POC) was fully activated at the highest emergency level [19] . Meanwhile, steady communication between the government and impacted sites was established [19] . News coverages and social media were used frequently to message residents the latest information and placement measures [19] . After the process of evacuation being accomplished, lots of shelters and goods were offered to evacuees to meet their basic requirements instantly by the government, volunteers and charitable organisms [19] . Meantime, professional firefighters and other first responders from a variety of countries and regions were staying behind to fight for controlling wildfire [19] . At the same time, a number of evacuees contacted with Alberta Health Services (AHS) to request for mental health support [19] . Eventually, the fire was under control thanks for the joint effort made by the Government of Alberta, Regional Municipality of Wood Buffalo, Canadian Red Cross, Indigenous communities, and the Government of Canada on July 4th [19] . Generally speaking, the emergency operations conducted by the government of Alberta was timely and effective. The evacuation order ensured the basic safety of individuals while the mental health support helped to make the public calm down. However, the high demand on mental health support revealed the lack of professional staff in this specific field. Therefore, more support for mental health care were needed from society.
Option for long-term recovery measurements and Recommendations
After controlling the wildfire, the government started to concern about the reentry and recovery in the Fort McMurray region [19] . During the time, air and water quality was evaluated firstly by the monitoring program of the Government of Alberta, along with the regional Municipality of Wood Buffalo and the Wood Buffalo Environmental Association [19] . Meanwhile, the provincial and municipal officers worked together to ensure the availability of essential services such as electricity in the area, then the re-entry program was implemented and re-cleaning was started to be conducted by residents [19] .
As the wildfire was diminished and many people returned to their homes, the recovery programs became an important step of next phase [19] . By Learning from the recovery works of past disasters, 5 categories in total are grouped up: people, economy, reconstruction, Environmental and mitigation [19] .
People having experienced a tragedy often show symptoms of stress and anxiety [19] . Thus, there is a need of short-term mental health support, such as consultant, and instant and long treatments [19] . In order to figure out and address the mental problem, the government has approved a budget of 18.65 million dollars for the first year to conduct mental health programs [19] . Moreover, in order to provide temporary shelters for homeless especially those who do not have ability to afford the rent, the Government of Alberta established some financial association to provide economical support [19] .
Suffering from the disaster, lots of small businesses faced challenges [19] . Not only lost their employees, they also had their properties and goods damaged. Nevertheless, many small businesses also played an important role in recovery work in communities [19] . A support of 1000 dollars was granted to each eligible small business by Canadian Red Cross [19] . The Regional Municipality of Wood Buffalo has been working on encouraging as much recovery work as possible to local contractors and local workers, and looking at promoting “buy local” or “open for business” campaigns [19] . Besides, employees cannot be ignored as well. The connection between employees and employers should be paid attention to. Moreover, as a fundamental and key role in economic development and recovery, the stability and availability of public transportation need to be ensured.
In terms of the policies about environment, the theme is to monitor the long-term environmental impacts and to mitigate potential risks [19] . To meet the requirement, the main work will include but is not limited to monitor air, soil and water quality [19] . In order to record the long-term environmental impacts of wildfire, the regional monitoring will be modified [19] . In fact, the environment contains not only air and water. What the government do is just to ensure the safety of basic living condition. However, what should be taken into account are not only the climatic factors, but also the non-climatic factors such as the disease spread rate. In addition, other pollutants also need monitoring in order to avoid any potential negative impacts on residents.
Even as the communities in the Fort McMurray area have started the clean-up and recovering from the disaster, lots of works are still required to reduce and mitigate the risk of future natural emergencies [19] . In order to reduce the frequency of disasters like wildfire, the government has been increasing the invest in mitigation of natural disasters and other public safety risks [19] . To achieve this goal, the Government of Alberta is committed to reduce and avoid the potential of other wildfire events that may threaten communities in the Regional Municipality of Wood Buffalo in the future [19] . Three programs designed for this project are named Disaster preparedness, FireSmart, and Flood mitigation respectively [19] . Probably these programs have not shown an obvious effect as people surviving from Fort McMurray Fire are highly alert to wildfire. However, it is quite wise and farsighted to launch such programs for long-term prevention.
Layering Perspectives: Other Alternatives Solutions and Recommendations
In this section we welcome contributions from scholars and students to widen the scope of possible solutions in addressing the impacts associated with wildfire. Those interested in contributing to this case study may use the following questions as a guide:
- Viewed through the lens of other disciplines and professions, what other actors become relevant when considering recovery recommendations and preventative strategies to address costs and impact associated with wildfires?
- What special expertise, resources, or theoretical orientations might others bring to help us better mitigate challenges associated with wildfires?
The 2016 Fort McMurray Fire is the largest, severest, and costliest natural disaster in Alberta’s, even the Canadian, history [1] . The occurrence of the wildfire is mainly due to the supportive natural factors, including the dry and hot summer environment in Alberta and the availability of plenty of fuels such as forests wood and oil sands [5] . The life of thousands of residents in the area and the local environment were greatly destroyed by the fire. The economic activities and social development of Fort McMurray were impacted and even paused in a long period as well.
Both the local and national government showed a timely response to the emergency right after it occurrence, taking a series of actions to ensure the security of local people and to control the fire. Nonetheless, a more completed emergency reacting system should be carried out by the government in order to prevent secondary injuries after the catastrophe. Also, a cooperative approach which involves both the government and the public is required to ensure the steady long-term efforts for the recovery programs of Fort McMurray in the future. The rationale of this solution is the intensity and severity of the negative impacts of the disaster. As discussed above, the 2016 Fort McMurray Fire is one of the severest and costliest natural disaster in Canadian history [1] . Although the wildfire only last for a few months, its huge damage and negative impacts cannot be eliminated in even several years. Thus, countless efforts need putting into the urban and environmental recovery, which is definitely cannot be accomplished only by the government. A holistic approach [20] [21] to involve the public into the post-disaster reconstruction will be the most effective and practical way in regard to the recovery program, which includes environmental recovery, community rebuilding, economy restarting, and mental health support for the disaster victims [19] . To be specific, the government should implement a set of policies to encourage more individuals and charitable organizations to take part in the reconstruction of Fort McMurray area. The recovery programs will be conducted efficiently only when the public actively participate in and contribute their effort to the reconstruction. And it is the only possible way for the government to fill the deficit between the need of resources for the post-disaster recovery and what the government can provide.
- ↑ 1.0 1.1 1.2 1.3 Wikipedia. (2017). 2016 Fort McMurray Wildfire. Retrieved from: https://en.wikipedia.org/wiki/2016_Fort_McMurray_Wildfire
- ↑ Wikipedia. (2017). Saskatchewan. Retrieved from: https://en.wikipedia.org/wiki/Saskatchewan
- ↑ 3.0 3.1 Natural resources Canada. (2016). Fire management-an attitude shift. Retrieved from: http://www.nrcan.gc.ca/forests/fire-insects-disturbances/fire/13157
- ↑ 4.0 4.1 4.2 4.3 4.4 4.5 4.6 4.7 4.8 Leahy, S. (2016). Canada wildfire-what are the environmental impacts? Retrieved from: https://www.theguardian.com/environment/2016/may/11/canada-wildfire- environmental-impacts-fort-mcmurray
- ↑ 5.0 5.1 5.2 Wikipedia. (2017). Athabasa oil sands. Retrieved from: https://en.wikipedia.org/wiki/Athabasca_oil_sands
- ↑ Government of Alberta. (2017). Facts and Statistics on Alberta energy. Retrieved from: http://www.energy.alberta.ca/OilSands/791.asp
- ↑ 7.0 7.1 7.2 The Conference Board of Canada. (2016). Economic impacts of the Fort McMurray wildfires. Retrieved from: http://www.conferenceboard.ca/press/newsrelease/16- 05-17/economic_impacts_of_the_fort_mcmurray_wildfires.aspx
- ↑ Government of Canada. (2016). Boreal Plains Ecozone. Retrieved from Ecological Framework of Canada: http://ecozones.ca/english/zone/BorealPlains/land.html
- ↑ Government of Canada . (2017). Canadian Climate Normals 1981- 2010 Station Data. Retrieved from Government of Canada: http://climate.weather.gc.ca/climate_normals/results_1981_2010_e.html?stnID=2519 &lang=e&province=AB&provSubmit=go&page=51&dCode=1
- ↑ 10.0 10.1 Warzecha, M. (2016). “Why the boreal burns: The trees surrounding Fort McMurray are hard-wired for fire.” Retrieved from National Post: http://news.nationalpost.com/news/canada/why-the-boreal-burns-the-trees- surrounding-fort-mcmurray-are-hard-wired-for-fire
- ↑ Lucas, P. (2016). Fort McMurray wildfire burning so hot, only weather can stop it. Retrieved from CBC news: http://www.cbc.ca/news/technology/alberta-wildfire-science-background-1.3565932
- ↑ Daryl M. Wightman, S. G. (1997). The Lower Cretaceous (Aptian) McMurray Formation: An Overview of the Fort McMurray Area, Northeastern, Alberta. Retrieved from CSPG Special Publications: http://archives.datapages.com/data/cspg_sp/data/018/018001/312_cspgsp0180312.ht m
- ↑ Frances J. Hein, D. K. (2006). The Athabasca Oil Sands — A Regional Geological Perspective, Fort McMurray Area, Alberta, Canada. Natural Resources Research, 15, 85-102. Retrieved from https://link.springer.com/article/10.1007/s11053-006-9015-4
- ↑ Powers, L. (2016). CBC News Technology & Science. Retrieved from CBC: http://www.cbc.ca/news/technology/alberta-wildfire-science-background- 1.3565932
- ↑ Government of Alberta. (2015). Fort McMurray Mineable Oil Sands IRMP. Retrieved from Alberta Environment and Parks: http://aep.alberta.ca/lands-forests/landuse-planning/fort-mcmurray-mineable-oil- sands-irmp.aspx
- ↑ Alberta Canada. (2016). About the industry. Retrieved from Alberta Canada: http://www.albertacanada.com/business/industries/og-about-the-industry.aspx
- ↑ Worland, J. (2016). Wildfire in Fort McMurray, Canada Causes Oil Prices to Surge. Retrieved from Time: http://time.com/4319287/fort-mcmurray- canada-wildfire-oil-prices/
- ↑ 19.00 19.01 19.02 19.03 19.04 19.05 19.06 19.07 19.08 19.09 19.10 19.11 19.12 19.13 19.14 19.15 19.16 19.17 19.18 19.19 19.20 19.21 19.22 19.23 19.24 19.25 19.26 19.27 19.28 19.29 19.30 Government of Alberta. (2017). Home Again: Recovery after the Wood Buffalo Wildfire. (Report No. ISBN 978-1-4601-3135-0). Retrieved from https://www.alberta.ca/documents/Wildfire-Home-Again-Report.pdf
- ↑ Oshry, Barry. (2008). Seeing Systems: Unlocking the Mysteries of Organizational Life , Berrett-Koehler
- ↑ Auyang, Sunny Y. (1999). Foundations of Complex-system Theories: in Economics, Evolutionary Biology, and Statistical Physics , Cambridge University Press
Post image: Wild fire spreads in Fort McMurray. Public domain, via Wikimedia Commons
share this!
May 10, 2024
This article has been reviewed according to Science X's editorial process and policies . Editors have highlighted the following attributes while ensuring the content's credibility:
fact-checked
peer-reviewed publication
trusted source
Wildfires in old-growth Amazon forest areas rose 152% in 2023, study shows
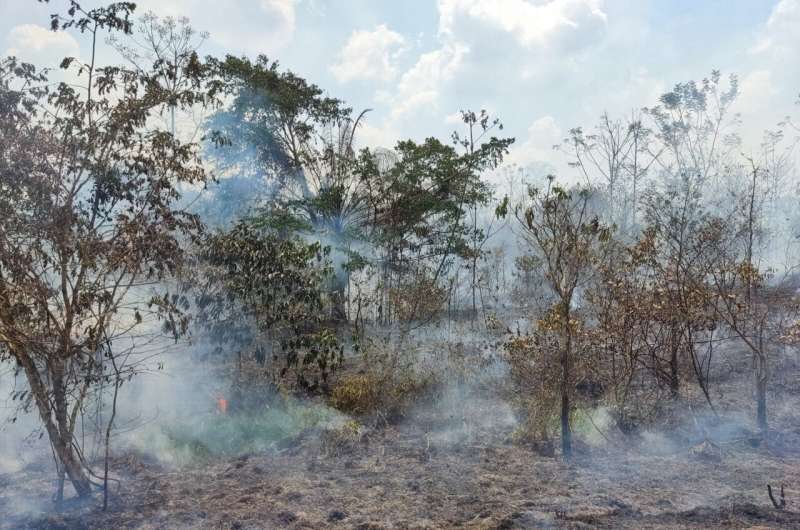
Although the rate of deforestation in the Brazilian Amazon fell in 2023, the region is faced with another challenge in the shape of fire affecting the native vegetation that has so far been spared destruction. An article published in the journal Global Change Biology warns that wildfires in old-growth forests rose 152% last year compared with 2022, despite a drop of 16% in the total number of fires throughout the Amazon and a 22% drop in deforestation.
In an analysis of satellite images, the authors detected a rise in forest wildfires from 13,477 in 2022 to 34,012 in 2023. The main cause was drought. The region has been experiencing longer and more frequent dry periods. There were prolonged droughts in 2010 and 2015-16, which left the forest more prone to fire and led to fragmentation of the plant cover. Another severe drought began last year and is still in progress, making the situation still worse.
According to surveys by the National Space Research Institute (INPE), the number of fires throughout the Amazon in the first three months of 2023 was 7,861, more than in any of the previous eight years and more than half the Brazilian total (followed by the Cerrado, with 25%). Until then, the highest first-quarter number recorded had been 8,240 (in 2016).
"It's important to understand the geographic pattern of these fires. Each of the areas concerned requires a different response. Our analysis pointed to more fires in old-growth forest areas than in previous years, which is alarming not just because of the loss of vegetation, which is invariably followed by deforestation, but also because the carbon stored by the forest becomes carbon emissions when it burns," said Guilherme Augusto Verola Mataveli, corresponding author of the article and a remote sensing specialist with INPE's Earth Observation and Geoinformatics Division.
Last year, some members of the research group published another article showing that wildfires increased along an emerging deforestation frontier in the area of Boca do Acre in the southwest of Amazonas state, North Brazil, between 2003 and 2019.
"Old-growth forest stores larger amounts of carbon, which becomes greenhouse gas emissions when it burns, contributing to climate change. Another negative effect relates to public health problems. In October 2023, Manaus [the capital of Amazonas state] had the worst air quality of any city in the world bar one," Mataveli said.
Burnings also increased in other states, including Pará, where the number of old-growth forest fires reached 13,804 in 2023, up from 4,217 in 2022.
The situation in Roraima is one of the worst in the region: Over half the fires detected in the Amazon in 2024 have occurred in this state, which has the fifth-largest Indigenous population in Brazil (97,320) and saw 14 of its 15 municipalities declare a state of emergency in March because of fire. Schools were closed because of the smoke, and severe drought left Indigenous communities without access to food and exposed to respiratory disorders, among other problems.
In response to Agência FAPESP, the National Center for Forest Fire Fighting and Prevention (PREVFOGO), an arm of IBAMA, the main federal environmental agency, said it has been working with other institutions since November 2023 to combat and prevent forest fires in Roraima. More than 300 firefighters and four aircraft have been involved in this campaign since January.
"Climate change is a key driver of the increase in forest fires, and El Niño has also added risk owing to its links with the prolonged drought in the region. We stress the importance of the firefighting efforts of state and municipal environmental authorities in collaboration with federal agencies. This partnership is fundamental to assure strategic and effective prevention of forest fires ," the IBAMA/PREVFOGO statement said.
The Ministry of Environment and Climate Change (MMA) responded to a request for a statement by reinforcing the above points.
Forest resilience
Tree mortality due to fire in primary forest areas often exceeds 50% of the above-ground biomass, so wildfires can greatly reduce the volume of carbon stored in the Amazon in the long term.
In February, carbon emissions due to fire in Brazil were the highest for 20 years, reaching 4.1 megatons, with Roraima in the lead, according to the Copernicus Atmospheric Monitoring Service. Copernicus is the Earth observation component of the European Union's space program.
Forest resilience is also weakened by wildfires, affecting the forest's capacity to create a humid microclimate below the canopy that contains and recycles moisture within the ecosystem.
Another point made by the researchers in the article is that the increase in invasive wildfires due to heightened forest flammability poses a significant challenge to traditional subsistence farmers who normally use controlled fire as a land management strategy.
According to Luiz Aragão, leader of the research group and last author of the article, "The Amazon is becoming more vulnerable environmentally, socially and economically as time passes without effective solutions to the fire problem." Although deforestation rates have fallen lately, the area affected continues to expand.
"We predicted this in an article by our group published in 2010 in the journal Science ," Aragão said. "Both deforested areas and areas where the forest is now being destroyed are active sources of fire ignition by humans. Deforestation fragments the landscape, creating more boundaries between forest areas and open areas and making old-growth forest areas more permeable to fire."
"The aggregate impact of extreme droughts like the current one, alongside landscape fragmentation, continuous use of fire, more areas of fire-degraded forest, illegal logging and edge effects will make the forest increasingly flammable. Urgent measures are needed to mitigate fires and maintain the Amazon as Brazil's greatest asset to achieve sustainable national development."
The article also advocates more command-and-control operations, more numerous and better-equipped fire brigades, and constant improvement of monitoring systems.
"With the use of artificial intelligence, we can try to develop systems that not only show where fires are occurring but also predict where they're most likely to break out in future so that we can focus preventive action on specific areas," Mataveli added.
Journal information: Global Change Biology , Science
Provided by FAPESP
Explore further
Feedback to editors

New study examines the price tag of phasing-out coal
19 minutes ago

Researchers breed tomato plants that contain the complete genetic material of both parent plants
22 minutes ago

Dice snakes found to use a variety of techniques to more effectively fake their own deaths
27 minutes ago

New surface acoustic wave techniques could lead to surfing a quantum internet
30 minutes ago

Researchers reveal how genetically identical water fleas develop into different sexes

Research shows that 'softer' proteins can cross into the nucleus quicker
39 minutes ago

Smaller laser facility uses new method to exceed previous record for proton acceleration

Possible new process for the synthesis of rare nuclei in the universe
49 minutes ago

Scientists discover huge magnetic toroids in the Milky Way halo
53 minutes ago

Researchers create complex quantum graph states with photons
54 minutes ago
Relevant PhysicsForums posts
The secrets of prof. verschure's rosetta stones.
May 9, 2024
A very puzzling rock or a pallasite / mesmosiderite or a nothing burger
May 8, 2024
M 4.8 - Whitehouse Station, New Jersey, US
What is global warming due to, large eruption at ruang volcano, indonesia.
May 2, 2024
Tidal friction and global warming
Apr 20, 2024
More from Earth Sciences
Related Stories

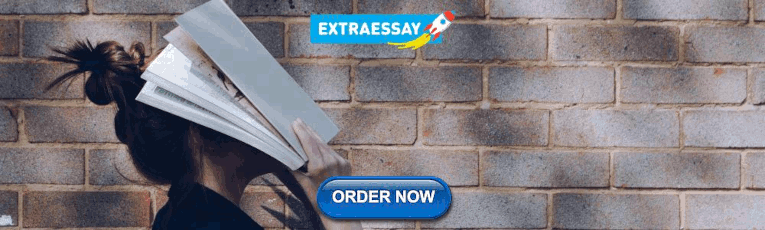
Wildfires in wet African forests have doubled in recent decades, large-scale analysis finds

Nearly 3,000 fires in Brazilian Amazon in February, new record
Feb 29, 2024

Number of fires in the Brazilian Amazon in August-September 2022 was highest since 2010
Feb 14, 2023

Smoke from Brazil Amazon rainforest fires suffocates Manaus
Oct 14, 2023

Forest fires in Bolivia consume vast area: official
Aug 8, 2021

Fires increase in Brazilian Amazon in July
Aug 1, 2022
Recommended for you

Weaker ocean currents lead to decline in nutrients for North Atlantic ocean life during prehistoric climate change
May 11, 2024

Clues from deep magma reservoirs could improve volcanic eruption forecasts

New 'forever chemical' cleanup strategy discovered

Biogeographical evidence shows trickster animal folklore is limited by environmental factors

Improved wildfire smoke model identifies areas for public health intervention

Net zero plans show limited climate ambition on 'residual' emissions
Let us know if there is a problem with our content.
Use this form if you have come across a typo, inaccuracy or would like to send an edit request for the content on this page. For general inquiries, please use our contact form . For general feedback, use the public comments section below (please adhere to guidelines ).
Please select the most appropriate category to facilitate processing of your request
Thank you for taking time to provide your feedback to the editors.
Your feedback is important to us. However, we do not guarantee individual replies due to the high volume of messages.
E-mail the story
Your email address is used only to let the recipient know who sent the email. Neither your address nor the recipient's address will be used for any other purpose. The information you enter will appear in your e-mail message and is not retained by Phys.org in any form.
Newsletter sign up
Get weekly and/or daily updates delivered to your inbox. You can unsubscribe at any time and we'll never share your details to third parties.
More information Privacy policy
Donate and enjoy an ad-free experience
We keep our content available to everyone. Consider supporting Science X's mission by getting a premium account.
E-mail newsletter
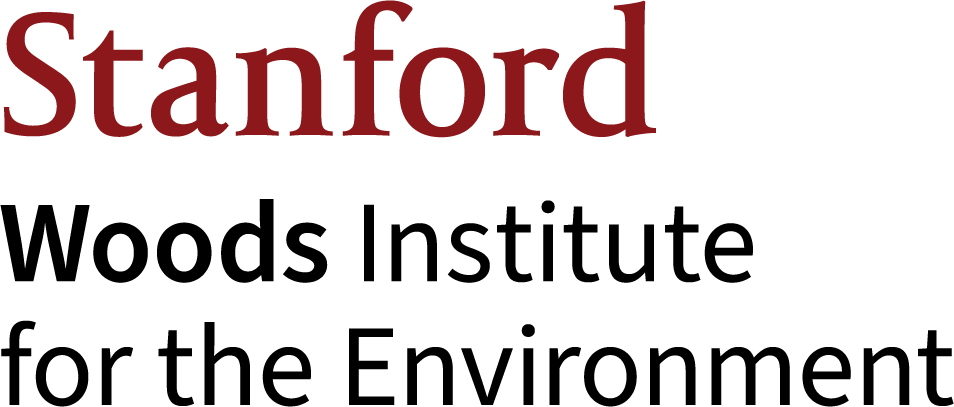
Wildfire: Assessing and quantifying risk exposure and mitigation across western utilities

In the Western United States, wildfires ignited by electric infrastructure have caused devastating damage and created billions of dollars in liability, throwing finances into question for utilities such as Hawaiian Electric Company (HECO), Pacific Gas & Electric (PG&E) in California, and PacifiCorp in Oregon. Wildfires, made more severe by climate change, threaten areas beyond those in the West that have recently experienced catastrophic fires. This increasing exposure to wildfire risk puts utility finances in jeopardy and, in turn, impedes the construction of electric infrastructure needed for a transition to renewable energy. A new white paper by researchers at Stanford’s Climate and Energy Policy Program analyzes Western investor-owned utilities’ wildfire mitigation plans, highlighting those that are leading the way and identifying steps utilities with exposure to wildfire risk should be taking. The researchers emphasize the importance of creating such plans and sharing them with the public to inform better decision-making by utilities, regulators, and investors.
Read the white paper here
Media Contacts
Christine black, explore more.

How to get corporate sustainability right
Mastercard’s chief sustainability officer discusses how companies can avoid greenwashing and align with their social and environmental values.
- Event Coverage

Gas stoves spread harmful pollution beyond the kitchen, study finds
Testing done in a Harlem, NY neighborhood and across the country reveals that people who own gas and propane stoves inhale toxic amounts of nitrogen dioxide, according to Rob Jackson's study.
- In the Media

Gas Stove Pollution Risk Is Greatest in Smaller Homes, Study Finds
Rob Jackson's recent publication warns against gas and propane stoves as they emit an asthma-inducing pollutant, nitrogen dioxide, around the house.

Want to create or adapt books like this? Learn more about how Pressbooks supports open publishing practices.
Case Study: Climate Change and the Risk of Wildfires in Canada
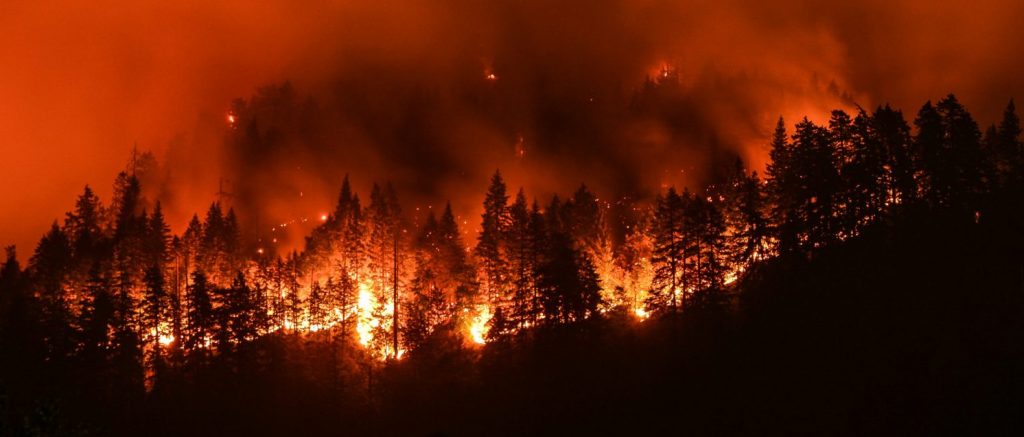
The year 2021 was one of Canada’s busiest fire seasons in recent history according to Natural Resources Canada. The fire season started early with dry spring conditions in the prairies. From March to May, communities in Alberta, Saskatchewan and Manitoba received evacuation alerts and orders. British Columbia, Saskatchewan, Manitoba, and Ontario all experienced fire seasons well above the average. As of September 15, 2021, there were 6,224 wildfires in Canada that burned an area of 4.18 million hectares, more than three fourths the size of the province of Nova Scotia, compared to a 10-year national average of about 5,348 fires and 2.59 million hectares (National Wildland Fire Situation Report, 2021 [1] ).
On June 29, 2021, for a third consecutive day, Lytton, a tiny village of about 250 residents in British Columbia, broke Canada’s national heat record with an all-time high temperature of blistering 49.6C. The next day, 90% of the village was obliterated by flames in minutes as more than a dozen wildfires raged in British Columbia amid the most extreme heat wave recorded in the Canadian Pacific Northwest. That was British Columbia’s third worst fire season in terms of area burned behind 2018 and 2016 with the death toll ranging in the hundreds.
From July to August 2021, forest fires blazed through northwestern Ontario forcing thousands of First Nation people to flee their communities in Northern Ontario. In 2021, Ontario saw an exponential surge in wildfires that, at 1,198, were more than double the 10-year average of 520 wildfires. Over 793,000 hectares of land were burned, an area larger than the Greater Toronto Area (GTA) and more than five times the annual average of about 153,000 hectares
According to Natural Resources Canada, climate change will affect our forests in multiple ways. Some effects will be sudden and dramatic while others will be gradual and subtle. Global warming and forest fires are linked in a vicious cycle: warmer temperatures increase the likelihood of fires and burning forests release greenhouse gasses in the atmosphere that worsen global warming. In British Columbia for example, extreme wildfire years in 2017 and 2018 each produced three times more greenhouse gases than all other sectors of the province combined. In the words of Dr. Mike Flannigan, a professor with the Department of Renewable Resources at the University of Alberta who has researched wildland fires for decades, our “future is smoky.” [2]
Canadian wildland fire management agencies are tasked with managing wildland fires. Wildland fire management includes fire preventions, mitigations, detection, and response to forest fires. The annual national cost of forest fire protection has increased by about $150 million per decade since data collection started in 1970 reaching close to 1.5 billion dollars in 2017 or about $40 person.
The questions below are intended to deepen your understanding of the threat of wildfires and their associated costs. For each question, there are resources that you can use as a starting point for your research, but you are not limited to these resources. In a paragraph or two, provide an answer to each question that is well supported by the evidence you collected (i.e., data and articles).
- How costly are wildfires for residents, businesses, critical infrastructure, and wildland fire management agencies?
Resource: Government of Canada, Cost of wildland fire protection (nrcan.gc.ca)
- For a province of your choice, find how frequent the wildfires are. What are the province’s major fire risk areas and major historical wildfires?
Ontario Ministry of Natural Resources and Forestry, Forest Fire Info Map (gov.on.ca)
BC Wildfire Service, BC Fire Atlas
BC Wildfire Service, BC Major Historical Wildfires
- How are wildfires and climate change related?
Resource: How wildfires affect climate change — and vice versa (theconversation.com)
- How will the rising risk of wildfires affect our ability to purchase fire damage coverage for our homes and properties and the solvency of insurance companies?
Insurance Bureau of Canada, Wildfire insurance coverage
Insurance company overwhelmed by cost of California wildfire, goes out of business
Watch “ State takes over Merced insurance company unable to pay out claims after Camp Fire ”
- “National Wildland Fire Situation Report,” Natural Resource Canada, accessed October 20, 2021, https://cwfis.cfs.nrcan.gc.ca/report ↵
- “Forest Fires and Climate Change,” Climate Atlas of Canada, accessed August 30, 2021, https://climateatlas.ca/forest-fires-and-climate-change ↵
Module 1: What Is Risk? Copyright © by Tsvetanka Karagyozova is licensed under a Creative Commons Attribution-NonCommercial-ShareAlike 4.0 International License , except where otherwise noted.
Share This Book
Wildfires in recent years have reversed some of the progress made in eliminating air pollution, new study suggests
Much of the progress made since the start of the century has been reversed.
The smoke from wildfires in recent years has been so intense that it is decreasing air quality in the majority of the U.S., reversing some of the improvements made to air quality in the last several decades, according to new research.
Wildfire smoke in the U.S. had a "notable influence" on recent air quality trends in nearly 75% of states in the continental U.S., despite air quality that has been steadily improving over the past several decades, largely due to policies like the Clean Air Act , according to a study published in Nature on Wednesday.
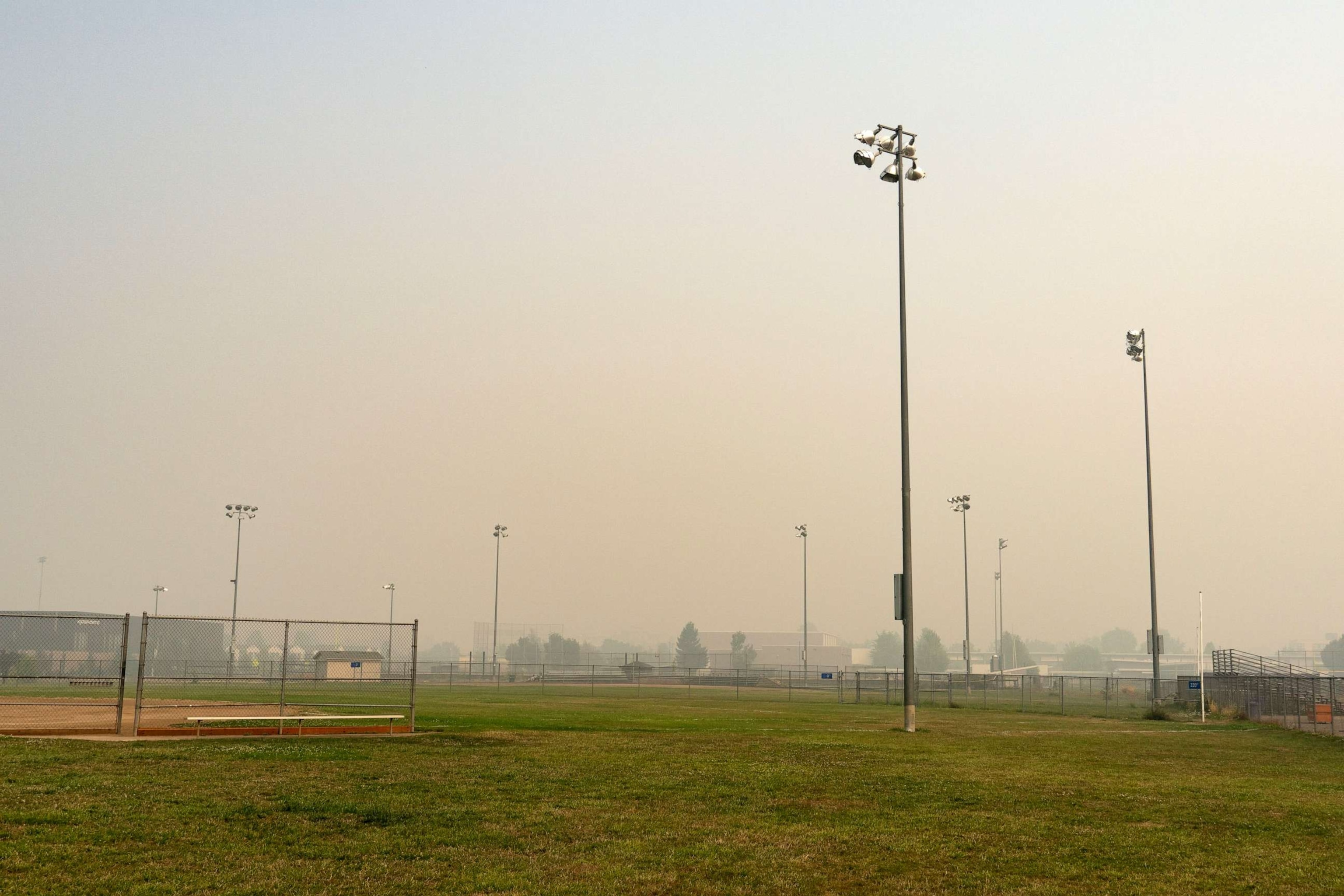
Wildfires have been increasing in size, frequency and intensity in recent years -- at the same time that progress in air quality improvement has stagnated across much of the U.S., with some regions reporting rising levels of fine particulate matter (PM2.5), the study found.
MORE: New app will allow New Yorkers to avoid commuting through the poorest air quality
The biggest surprise for the researchers was the extent of the influence of wildfires on diminishing air quality throughout the country, even reversing the progress made in recent decades in some regions, Marshall Burke, associate professor at Stanford University's Doerr School of Sustainability and lead author of the study, told ABC News.
"What we found was a pretty substantial and widespread fingerprint of wildfires on air pollution trends in the U.S.," he said.
MORE: Record-breaking wildfires have occurred all over the Northern Hemisphere during 2023, new report finds
Researchers combined air pollution data from ground and air sensors between 2000 and 2022 to determine the contribution of wildfire smoke to PM2.5 trends and found pollution was declining in 41 of the 48 states before 2016.
That decline has since slowed in 31 states and begun to reverse in 11 states, according to the paper.
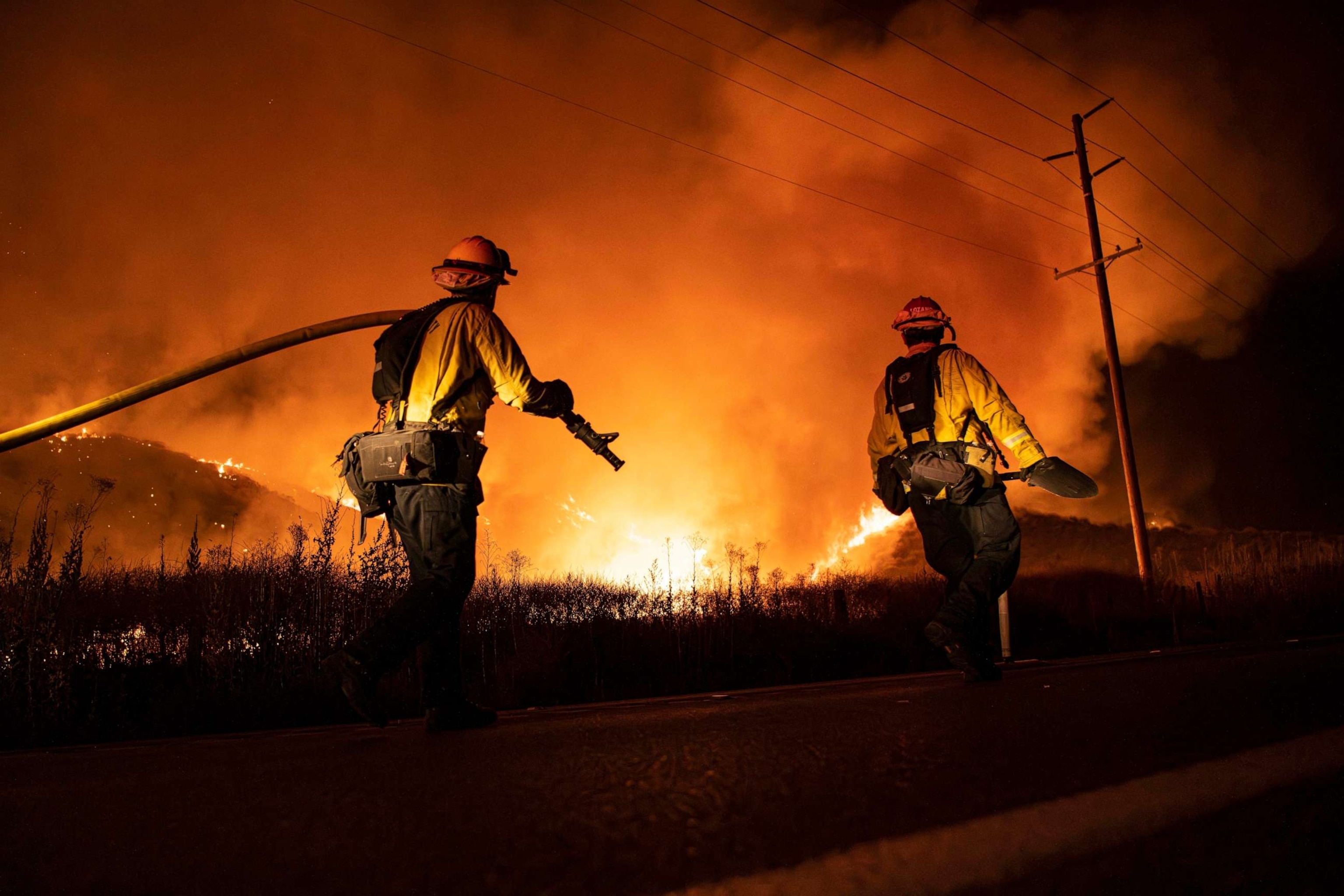
Smoke from just six years of wildfires in North America, from 2016 to 2022, was found to have eroded nearly 25% of the air quality progress made in the U.S. since the start of the century, Burke said.
"All of this success we'd had before is being rapidly unraveled, at least in some parts of the country, by wildfires," he said. "And that's a that's a concern."
MORE: Canada breaks record for annual wildfire smoke emissions
Fine particulate matter , known as PM2.5, is 30 times smaller in diameter than a human hair and cannot be seen by the naked eye. Exposure to concentrated amounts of PM2.5 can cause both short-term effects such as irritation of the eyes, nose and throat; coughing, sneezing; and shortness of breath, and long-term effects such as worsening of conditions such as asthma and heart disease, according to the U.S. Environmental Protection Agency .
The severity of the wildfires in recent years are a result of a combination of a century of fire suppression, which has left more fuel on forest floors to ignite, and global warming, which is creating drier landscapes that amplify a spark of flames, Burke said.
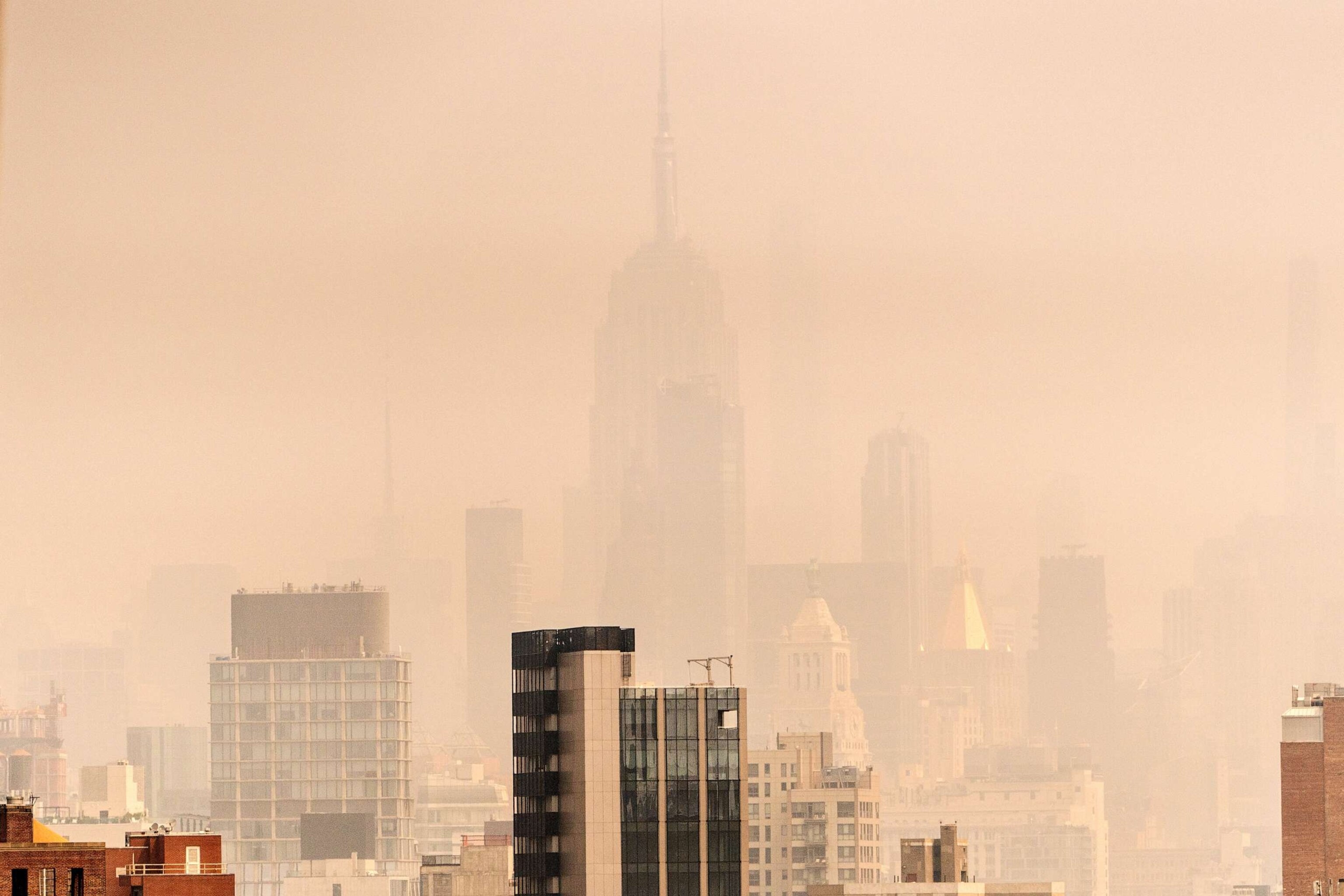
The study data doesn't even include the wildfires that have been burning in 2023, including the unprecedented wildfire season in Canada , Burke said.
While the West Coast has been dealing with acute events of dangerous air quality in recent years, the Northeast and even some parts of the South experienced toxic levels of air pollution in June.
MORE: Air quality concerns in US will continue through summer due to Canada's wildfires
More of these extreme air pollution events could continue in the future if severe wildfires continue to burn unabated, Burke said.
"I would be surprised if we didn't see more events analogous to this in future years," he said.
Related Topics
- Environment
Top Stories
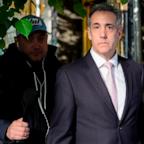
Trump trial updates: Michael Cohen describes efforts to catch and kill stories
- 7 minutes ago
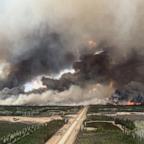
Canadian wildfire smoke prompts air quality alert in Midwest US
- 2 hours ago

Controversy follows Gov. Kristi Noem as she is banned by two more South Dakota tribes
- May 11, 6:02 PM
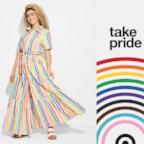
Target will only sell Pride Month collection in some stores after backlash in 2023
- May 10, 7:33 AM
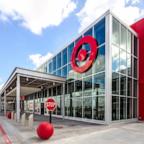
Woman uses self-checkout to steal $60,000 of items from same Target store over a year
- May 10, 5:03 AM
ABC News Live
24/7 coverage of breaking news and live events
- Search Menu
- Advance articles
- Browse content in Biological, Health, and Medical Sciences
- Administration Of Health Services, Education, and Research
- Agricultural Sciences
- Allied Health Professions
- Anesthesiology
- Anthropology
- Anthropology (Biological, Health, and Medical Sciences)
- Applied Biological Sciences
- Biochemistry
- Biophysics and Computational Biology (Biological, Health, and Medical Sciences)
- Biostatistics
- Cell Biology
- Dermatology
- Developmental Biology
- Environmental Sciences (Biological, Health, and Medical Sciences)
- Immunology and Inflammation
- Internal Medicine
- Medical Microbiology
- Medical Sciences
- Microbiology
- Neuroscience
- Obstetrics and Gynecology
- Ophthalmology
- Pharmacology
- Physical Medicine
- Plant Biology
- Population Biology
- Psychological and Cognitive Sciences (Biological, Health, and Medical Sciences)
- Public Health and Epidemiology
- Radiation Oncology
- Rehabilitation
- Sustainability Science (Biological, Health, and Medical Sciences)
- Systems Biology
- Browse content in Physical Sciences and Engineering
- Aerospace Engineering
- Applied Mathematics
- Applied Physical Sciences
- Bioengineering
- Biophysics and Computational Biology (Physical Sciences and Engineering)
- Chemical Engineering
- Civil and Environmental Engineering
- Computer Sciences
- Computer Science and Engineering
- Earth Resources Engineering
- Earth, Atmospheric, and Planetary Sciences
- Electric Power and Energy Systems Engineering
- Electronics, Communications and Information Systems Engineering
- Engineering
- Environmental Sciences (Physical Sciences and Engineering)
- Materials Engineering
- Mathematics
- Mechanical Engineering
- Sustainability Science (Physical Sciences and Engineering)
- Browse content in Social and Political Sciences
- Anthropology (Social and Political Sciences)
- Economic Sciences
- Environmental Sciences (Social and Political Sciences)
- Political Sciences
- Psychological and Cognitive Sciences (Social and Political Sciences)
- Social Sciences
- Sustainability Science (Social and Political Sciences)
- Author guidelines
- Submission site
- Open access policy
- Self-archiving policy
- Why submit to PNAS Nexus
- The PNAS portfolio
- For reviewers
- About PNAS Nexus
- About National Academy of Sciences
- Editorial Board
- Journals on Oxford Academic
- Books on Oxford Academic

Article Contents
Introduction, three catastrophic fires in the past five years, wildland–urban interface risk and climate change, discussion and recommendations: improving wildfire risk reduction efforts, author contributions, data availability statement, wildfire risk management in the era of climate change.

Competing Interest: The authors declare no competing interest.
- Article contents
- Figures & tables
- Supplementary Data
Costas Emmanuel Synolakis, Georgios Marios Karagiannis, Wildfire risk management in the era of climate change, PNAS Nexus , Volume 3, Issue 5, May 2024, pgae151, https://doi.org/10.1093/pnasnexus/pgae151
- Permissions Icon Permissions
The August 8, 2023R Lahaina fire refocused attention on wildfires, public alerts, and emergency management. Wildfire risk is on the rise, precipitated through a combination of climate change, increased development in the wildland–urban interface (WUI), decades of unmitigated biomass accumulation in forests, and a long history of emphasis on fire suppression over hazard mitigation. Stemming the tide of wildfire death and destruction will involve bringing together diverse scientific disciplines into policy. Renewed emphasis is needed on emergency alerts and community evacuations. Land management strategies need to account for the impact of climate change and hazard mitigation on forest ecosystems. Here, we propose a long-term strategy consisting of integrating wildfire risk management in wider-scope forest land management policies and strategies, and we discuss new technologies and possible scientific breakthroughs.
Here, we draw from research on wildland–urban interface (WUI) fires from forestry and climate science to emergency management and economics, and we summarize the complexity and offer recommendations for policy making. Its significance stems from the analysis of emergency response in recent deadly fires, and our integrated approach which brings together multiple, diverse disciplines to explore policy-related, strategic, and operational approaches for addressing wildland fire risk in the era of climate change.
Wildland fires have existed since the beginning of history, with Paleontological evidence suggesting that early forest fires started to spread about 383 million years ago ( 1 ). As humanity evolved, forest fires were allowed to burn, depending on the objectives of human settlers ( 2 ). For example, even with the advent of fire suppression capabilities in the late 19th century, fire control efforts in the United States focused around settlements, and those beyond were left to burn unabated ( 3 ).
Despite impressive advances in forestry, fire science and firefighting technologies, and the leaps in wildland firefighting capabilities compared to 200 years ago, wildfire deaths are on the rise. The early February 2024 wildfires in Valparaiso, Chile killed over 130 persons and were the deadliest natural disaster in the country since the 2010 Mw∼8.8 earthquake and tsunami ( 4 ) and occurred in what has been called a “megadrought” ( 5 ). The August 8, 2023 Maui fires resulted in the highest death toll in the United States since the 1918 Cloquet and Moose Lake fires, and they amount to the fifth deadliest incident on record nationwide ( 6 ). It has been argued that at least some of these casualties might have been prevented had there been evacuation orders or emergency alerts ( 7 , 8 ). Less than two months after this catastrophe in Hawaii, the nationwide test of the Integrated Public Alert and Warning System established by the Federal Emergency Management Agency (FEMA), a state-of-the-art capability which has served as a model for countries around the world, came as a stark reminder of the complexity of wildfire risk.
The National Academies of Sciences, Engineering, and Medicine ( 9 ) point out that wildfire smoke can be transported over fairly long distances downwind and contaminate indoor and outdoor air, indoor surfaces, soil, and surface and even drinking water. Air pollution by plume transport is an insidious yet potent source of morbidity and mortality. In Europe alone, Kollanus et al. ( 10 ) estimated 1,080 premature deaths in 2008 from wildfire-originated fine particles in the atmosphere, with southern and eastern Europe disproportionately affected. The 2018 Camp Fire (Butte County, CA) resulted in PM 2.5 concentrations in downtown San Francisco, 240 miles away, peaking about six times higher than, and about double the US benchmark, for two weeks after the event ( 11 ). Chen et al. ( 12 ) found that fine particulate matter from wildfires was significantly associated with increased cardiovascular and respiratory mortality at a global level, and estimated the associated death toll at 33,000, or approximately 0.6% of all deaths in 749 cities in 43 countries and regions, in 2000–2016.
The devastation caused by many wildfires does not come without nontrivial societal and political ramifications. Communities affected by wildfires face the trauma of death, injury, loss of livelihoods, damage to homes, and psychological effects. Notwithstanding the provision of relief aid, more often than not, decision-makers face the outrage of their constituents for everything they didn’t do, for example, issuing alerts and evacuation orders. Electorates tend to punish incumbents for wildfire deaths and damage, so there is scapegoating and denial of responsibility by officials, which makes forensic analysis exceptionally difficult.
Wildfire risk lies in the confluence of climate change and development in the WUI. According to the NASEM ( 9 ) report, the WUI in the United States has expanded by 50% in the past 50 years, the fastest growing land use type, and another 10% increase is projected by 2030. Stemming the tide of wildfire death and destruction will involve bringing together diverse scientific disciplines into policy. Here, we will first review three fires to elucidate challenges posed, then we discuss how climate change is projected to increase fire activity, that is the frequency and intensity of fires. We will explore how governments are responding, and we argue that improvements in fire suppression capabilities, although required in the short term, may be financially unsustainable in the long run.
Lahaina, Maui, HI
On August 8, 2023, fires broke out in different parts of Maui, Hawaii. One of the fires destroyed the historic town of Lahaina and claimed 98 lives, becoming the deadliest US wildfire in more than a century.
Maui is no stranger to vegetation fires. However, on August 8, dry vegetation and strong descending winds climbed up to about 125 kilometers per hour and caused the fire to quickly spread out of control. Firefighting resources were stretched thin, responding to multiple incidents on the island. Lahaina was ravaged, and the majority of its about 1,800 buildings were completely burned. Some people sought refuge at sea, whereas others burned in their cars while trying to evacuate ( 7 , 8 ).
As fires broke out, broadcast emergency messages and social media posts from the County of Maui provided updates, alerts, and evacuation calls for some areas but apparently not Lahaina, a densely populated area with limited escape routes. Confusing messaging and delayed evacuation alerts (if they even took place) did not help.
Looking at milestones in the timeline, around 3:30 PM, a major road was closed just north of Lahaina because of what appeared then as just a flare-up of an earlier fire. Maui County's social media page didn’t announce the closure until 4:46 PM Evacuation orders for the entire area appear not to have been issued when the road was closed, or even soon thereafter. Maui officials claimed that text alerts were sent out, but that power and cellular disruptions prevented residents from receiving them. Some survivors have reported receiving no warning messages before the fire reached them, whereas at least one visitor reported receiving an emergency alert on their cellphone at 4:17 PM, 47 minutes after the road closure. Many local residents reported that they didn’t know the flames were approaching until the fire was nearby, and some said that they were informed by neighbors to evacuate. By 5:19 PM the fire had reached the waterfront in Lahaina, and by 5:33 PM people were seeking shelter in the water.
All this is quite surprising. Not only was there a fire in Maui in 2018 with similar problematic issues, but Hawaii has one of the most sophisticated tsunami warning systems in the world, fine-tuned since 1946, when a 17-meter tsunami hit the islands in the aftermath of the 1946 Aleutian tsunami ( 13 ), claiming 159 lives. Sirens are an integral part of the islands’ warning capabilities, yet local authorities defended their decision not to activate them in this fire. Mechanical sirens, as in Hawaii's All-Hazard Statewide Outdoor Warning Siren System produce a single tone, alerting people of an impending hazard, but provide no information about the nature of the hazard, its severity and, most importantly, protective action guidance. Note that the siren system is referred to as all-hazard, including wildfires, and claimed as the largest in the world.
To be effective, sirens need to be associated with a single clear protective action ( 14 ). In fact, Maui County had advised people that “[w]hen a siren tone is heard other than a scheduled test, tune into local Radio/TV/Cable stations for emergency information and instructions by official authorities. If you are in a low-lying area near the coastline; evacuate to high grounds, inland, or vertically to the 4th floor and higher of a concrete building”. In other words, Maui residents were given incomplete information—the reference to evacuating to high ground is likely for tsunami emergencies, yet there is no reference in the instructions to tsunamis. This confusing messaging is exactly what emergency managers are taught to avoid yet, in the aftermath, local authorities claimed they couldn’t have done anything differently.
A High Wind Watch issued on August 6 spoke of gale-force winds from the northeast on Maui until the night of August 8, as Hurricane Dora passed south of the Hawaiian Islands. It was upgraded to a High Wind Warning for Maui on August 7, with forecasts of gale-force winds from the east. Fire danger in Hawaii is weather-dominated, as vegetation grows rapidly with subtropical rainfall extremes, and dries rapidly during drought periods ( 15 ). On August 8, dry conditions on the island, strong easterly winds, and densely populated areas on the west coast of the island combined to create a dangerous situation. With hindsight, this combination may have fulfilled the criteria for issuing a warning of potential extreme fire behavior.
The Maui disaster triggered political backlash and legal battles. State and national levels came under scrutiny over their handling of the situation. The Maui County Emergency Management Administrator resigned in the weeks following the disaster. Three months following the fire, the Governor announced the creation of a $150 million recovery fund to help those who were injured in the fires or lost family members if they waive their right to file associated legal claims. The fund is initially paid for by the State of Hawaii, Maui County, Hawaiian Electric, and Kamehameha Schools, all of which have been named in legal actions over the wildfires.
Mati, Greece
Emergency and civil defense managers in Hawaii should have known better. Five years earlier, almost to the day, on July 23, 2018, a fairly small brush fire claimed 104 lives in Mati, Greece. Mati is a seaside community of about 5,000, approximately 30 km west of Athens, and a popular summer destination. On July 23, very strong winds descending from Mount Penteli at about 95 km/h (gusting to over 110 km/h) pushed the fire rapidly over dry vegetation, causing it to spread quickly, covering one-half mile in about half an hour from the nearest highway east of the town to the sea. In total, the fire took two hours to spread from where it started to the nearest beach—there is security camera video that captured the ignition. About 1,200 buildings were destroyed by the fire.
People were burned in their cars as they attempted to flee, while hundreds of people escaped to nearby seaside cliffs, hiked down to the beach, and were evacuated hours later from the water. A large number of firefighting resources had already been committed to a major incident about 80 km to the east, leaving limited resources available for the Mati fire.
In Mati, there was no warning, and people self-evacuated when flames were hundreds of feet away or did so at the encouragement of neighbors. Some police officers and firefighters reportedly went door-to-door to urge people to evacuate, but the lack of evacuation planning led to a series of traffic management failures, including, in one case, leading evacuees back toward the fire. The government and civil defense denied responsibility and claimed they couldn’t have done anything differently, even with hindsight ( 16 ). Despite the rapid spread of the fire, our calculations using agent-based evacuation models showed that, with a proper evacuation plan and public warning, the area could have been evacuated in time.
Unlike Maui, the legal and political fallout of the Mati disaster had historic proportions. At first, there were ad hominem attacks against scientists who spoke out about the lack of evacuation orders as contributing to the high death toll. This is also what happened in several countries in the first months of the COVID pandemic—governments were shooting the messengers. Eventually, however, the Alternate Minister for Citizen Protection, the Secretary General for Civil Protection, the Chief of the Hellenic Fire Service and the Chief of the Hellenic Police resigned. A series of negligence lawsuits were filed against the government by those who had lost loved ones and/or their homes. In addition, a four-year criminal investigation culminated with a trial which began on October 31, 2022, with twentyone senior elected and appointed officials facing felony charges. On April 29, 2024, six of the twentyone defendants were found guilty of negligent homicide. Some have argued that the handling of the aftermath of this fire led to the government losing national elections a year later.
The new government that took over rolled out the 112 Emergency Communications Service, following our relentless advocacy, editorials in major newspapers, and actual implementation work. The new service integrates a unified, multi-agency public safety answering capability, based on the European emergency number 1-1-2 with a nationwide, integrated public alert and warning system. This system is now used by the General Secretariat for Civil Protection (i.e. the country's national emergency management agency) to deliver effective life-saving information to the public. Alert messages are delivered primarily through cell phone SMS and additional communications pathways. In addition, new legislation voted in 2020 established an all-hazards planning approach, and municipalities are required to prepare a single emergency operations plan with hazard-specific and function-based annexes, as required. An evacuation annex is now mandatory.
The Maui and Mati fires bear eerie similarities. Dry vegetation and high winds, which pushed the fires downslope to densely populated areas with poor evacuation routes, and insufficient firefighting resources combined to cause both fires to quickly spread out of control. Preparedness failures, poor warning capabilities, and an apparent deficiency in evacuation planning led to the high death tolls. In both locales, people were burned in their cars, in Mati some because of a lack of evacuation planning that resulted in drivers being redirected back to the inferno, while in Lahaina possibly because of delays in evacuation and confusion with closed roads. In both cases, officials claimed that call centers were overwhelmed. Both events had political repercussions, and in both cases officials abdicated responsibility, claiming they wouldn’t have done anything differently even with hindsight.
WUI fires need not be so ghastly.
Rhodes, Greece
On July 18, 2023, a fire broke out on the Greek island of Rhodes. The fire was initially confined to the island's mountainous center, but spread to the south on the fourth day, fanned by strong, northerly seasonal winds. By July 23, the fire had reached the southern coast of the island, scorching more than 16,000 ha. With multiple fires burning simultaneously throughout the country, resources were stretched thin, despite the reinforcements sent by EU countries and the United States. The island is one of the most popular tourist destinations in the Mediterranean, exceeding 2 million visitors every year, and roughly the same size as Maui.
When it comes to evacuations, visitors are a vexing challenge, not least because of their limited proficiency in the host country's language, and their lack of familiarity with the area they are visiting. Yet, Greek authorities were able to evacuate over 20,000 local residents and tourists from the affected areas in Rhodes, within half a day, making it Greece's largest ever wildfire evacuation ( 17 , 18 ). The country's public alert and warning system was used to send multiple evacuation messages, as the fire approached residential areas. The slower overall rate of spread of the Rhodes fire may have allowed for the timely evacuation. However, the scale of the evacuation was considerable for Greece, and much more so for an island like Rhodes, and we that without a public warning system and a tried emergency management program, such as 112, the outcome would have been quite different. Ultimately, the fire devastated homes, hotels, and other businesses, yet the only casualty was the tragic loss of a volunteer firefighter. The efficacious crisis management in the aftermath bears noting, as most evacuees were sheltered in hotels or hosted by locals from night one. While at first tourist arrivals drastically fell, and hundreds of incoming flights were canceled, normalcy was restored within three weeks.
The wildland–urban interface challenge
More people living in WUI areas enhance ignition opportunities. Moritz et al. ( 19 ) identified several socioecological linkages and have focused on the impact to ecosystems by and their interdependencies with humans. While fires in remote areas are usually triggered by lightning storms, most wildfires appear to be human-caused ( 20 ). It is therefore hardly surprising that hot spots of fire activity have been identified in the WUI in the United States ( 21 ), and 95% of wildfires in Europe are reportedly caused by human negligence or arson ( 22 ). Radeloff et al. ( 23 ) state that the number of houses within wildfire perimentes thas doubled since the 1990s, due to both increased number of houses and larger burned areas. The number of wildfire-related deaths worldwide in the last four decades, based on the global EM-DAT database ( 24 ) shows that 10% of the fires appear responsible for 78% of the deaths (Figure 1 ). Furthermore, the single deadliest fire each year was responsible for 65% of all the deaths in that year, on average. In other words, a small number of fires produce the vast majority of deaths, while eight out of the ten deadliest fires occurred in the WUI.

Wildfire deaths per year, 1970–2023 ( 24 ).
Fires in the WUI pose unique challenges to fire suppression and emergency response. First, whereas urban and wildland firefighting tactics are fundamentally different, WUI fire suppression inherently involves both in close proximity, and tactical incompatibilities may appear. Urban fires will typically have multiple exposures with a fixed source, and direct fire attack using water or foam is the norm. On the other hand, wildland firefighting techniques very often involve indirect attack ( 25 , 26 ). For example, a fireline may be used to interrupt fuel continuity or a backfire may be employed to deprive the fire of oxygen. In the WUI, exposure usually involves a long flame front and multiple sources of ignition. In WUI fires, structural firefighters operate in close proximity with their wildland counterparts, which often creates challenges in communications and coordination, especially when they come from different organizations and jurisdictions.
Different firefighting tactics also mean different equipment. Wildland firefighting personal protective equipment is lighter because structural and atmospheric hazards are more limited in the open, and mobility is essential ( 26 ). Wildland firefighting engines are typically smaller and carry less equipment to remain mobile. This essential mobility in the wilderness may become a limiting factor, and possibly a source of danger, to firefighters in the WUI.
In addition, delayed fire reporting or increased response times increase fire risk to isolated homes and other structures ( 27 ). Fireline access can be challenging, as small backcountry roads and covered bridges might constrain anything but the smallest fire engines, and mutual aid resources could find navigating poorly charted rural roads a hindrance. Also, water supply may be scarce because of a lack of fire hydrants or failures during a power outage ( 28 ). This was the case in Lahaina, in both 2018 and 2023 and in the 2024 Viña del Mar fires in Chile, which took place while this paper was under review.
Second, evacuations are often required in WUI fires but are substantially different than organized evacuations implemented before river floods, landslides, and other natural hazards, or following accidents involving hazardous materials. Wildfire evacuations usually are decided and implemented on very short notice, notably because of difficulties in forecasting weather and predicting fire behavior. In Australia, in particular, there was debate about “stay and defend or go”, but the discussion seems to have settled in favor of “go” ( 29 ). Emergency planning helps alleviate some of that uncertainty. A small number of evacuation courses of action may be analyzed and included in emergency plans, offering options depending on the relative position of residential areas and the fire perimeter, as well as the current and projected weather, topography, and fuels. In Portugal, for example, predesigned evacuation routes and shelter locations are communicated to the public before disaster strikes, thus reducing clearance times when evacuations are warranted ( 30 ).
Regardless, many WUI areas have poor evacuee egress options, not only because of narrow, winding roads, but also traffic congestion ( 31 ). For instance, 23 people, including three seasonal firefighters, were trapped and perished while evacuating the village of Artemida, Greece during the 2007 fire south of ancient Olympia ( 32 ), over a narrow backcountry road that was the only option ( 33 ). In the 2018 Camp Fire in Northern California, staff evacuated patients from the local hospital in their own vehicles. On one occasion, the drive to safety out of the heavily wooded area allegedly took about three hours. This was a very difficult terrain for timely evacuations, nonetheless over 40,000 managed to evacuate. Alerts were reportedly not received by at least 50% of the residents, but completely without them the death toll would likely have been much higher than the 84 who perished. In another example, serious traffic congestion ensued when residents in West Kelowna, British Columbia were asked to evacuate during the 2015 Munt Law wildfire ( 34 ).
With this inadvertently rapid operational tempo, reducing the time between decision-making and the actual evacuation by providing people advanced notice is paramount. Mati and Maui and, to a lesser extent, Paradise—all three incidents were haunted by the lack or failure of public alert and warning systems ( 35 ).
Third, wildfires can rapidly spread out of control and increase in complexity. Large fires are among the most complex incidents, not least because of the geographic area involved, the large number of resources involved, organizational complexity, jurisdictional boundaries, weather, and difficulty in predicting fire spread ( 36–39 ). WUI fires add more layers of complexity, due to the threat to life, property and critical infrastructures, as well as political sensitivities. For example, it has been reported that, during the Mati fires, politicians were calling the fire department asking for prioritization of resources to favor friends and relatives. Climate change is one of the factors increasing the potential for extreme fire behavior, therefore making firefighting less routine while making fires themselves more routine.
Fourth, wildland fires are intricately related to critical infrastructure and form complex feedback loops which lead to compound events ( 40 , 41 ). The risk to energy production and generation assets and the power grid increases with wildfire incidence. Power lines can ignite wildfires through a variety of mechanisms, and fires can damage power utility assets, causing widespread power outages ( 42 ). California's largest utility, Pacific Gas and Electric, pleaded guilty to manslaughter charges and filed for bankruptcy in early 2019, accepting responsibility for its downed power lines that triggered the 2018 Camp Fire, allegedly the costliest natural disaster in that year worldwide. Hawaiian Electric is currently facing lawsuits for alleged negligence in the Maui fires. On the other end, in Mati, the country's national electricity corporation steadfastly refused to entertain even the suggestion that one of its power lines near the point of ignition may have triggered the fire, and thus far has gotten away with it.
The cascading effects of wildland fires increase agent-generated demands and complicate response-generated demands ( 40 , 43 ). Almost any critical infrastructure asset may be affected by wildland fires, even water treatment plants. Fires can melt underground water pipes, thus affecting firefighting, and they can destroy communication towers and power substations. Operators then need to find workarounds or make temporary repairs to restore service to priority customers, typically other critical infrastructure systems. At the same time, governments need to work with operators and affected critical customers to provide backup solutions. A vicious circle ensues, as infrastructure damage delays response and short-term recovery efforts, which in turn slow down restoration and repair ( 44 ).
Climate change and wildfire risk
Wildland fires have an intricate relationship with climate change. Bowman et al. ( 45 ) estimated that wildland fires are responsible for 19% of the anthropogenic radiative forcing. Climate change does yield higher temperatures and drier conditions that prime the landscape for fires to catch and spread more easily. Flannigan et al. ( 46 ) estimate that fire seasons will last 20 days per year longer in the northern high latitudes by the end of the century. The IPCC Sixth Assessment Report ( 47 ) notes that fire weather is expected to increase in many parts of the world and, a 2-degree global warming scenario is projected to increase burned area globally by 35%. These estimates are corroborated by regional studies, which point to an increase in the number of human-induced wildfires, burned area, and wildfire risk ( 21 , 48–56 ).
These scenarios are hardly fictitious, as climate change is already increasing wildfire risk around the world. Fire-prone areas are extending poleward to areas previously unaffected. Jolly et al. (2015) found that, by 2013, fire seasons had lengthened in about one-quarter of the Earth's vegetated surface, resulting in an increase of the global average fire season length by 18.7%, compared to 1979. They also estimated that, between 1979 and 2013, the burnable area affected by longer fire weather seasons had doubled. Furthermore, the frequency of long fire-weather seasons increased across more than half of the global vegetated area between 1996 and 2013, compared with 1979–1996. Regional studies corroborate these global findings by showing an increase in the number of fires and the burned area during fire seasons, as well as in the size, extent, and frequency of large fires ( 49 , 56–61 ). Consensus is emerging that the conventional suppression-centered wildfire and forest management strategies applied so far no longer efficiently address megafires, variously defined, but usually as fires that burn over 40,000 hectares.
Furthermore, overnight burning events (OBE), defined as fires that burn through the night, have recently been changing what used to be a familiar diurnal fire cycle. Luo et al. ( 62 ) found that 20% of the 1084 fires burning over 1000 hectares in the period 2017–2020 were OBEs, peaked in the summer, and were primarily associated with droughts. Balch et al. ( 50 ) claimed that, worldwide over the past 30 years, flammable nights have increased by seven, across all burnable lands, and found that night fires are about 7% more intense, while the vapor pressure deficit has increased by 25%. For the western United States, they estimated an 11-day increase over the same period. There is little doubt that there has been an acceleration of OBEs.
Figure 2 depicts megafires between 2012 and 2022 in Europe, Canada, and the United States, compiled out of three different datasets. The European Forest Fire Information System is operated by the European Commission Joint Research Center. Burned areas are estimated from satellite imagery, and information was available until 2023. Natural Resources Canada maintains the Canadian Wildland Fire Information System, based on a compilation of information provided by Canadian fire management agencies including provinces, territories, and Parks Canada. At the time of this writing, data was available only until 2021. In the United States, the National Interagency Fire Center Open Data Site provides a wealth of wildfire-related geospatial information. This dataset includes wildland fires reported to the Integrated Reporting of Wildland Fire Information incident service until 2023.

Megafires in Europe ( 63 ), Canada ( 64 ) and the United States ( 65 ) between 2012 and 2022.
One conclusion from the map in Figure 2 is the lack of a unified system for reporting wildfires around the world. Wildfire data collection around the world is a highly localized endeavor. Data collection techniques vary widely, from the compilation of reports from fire services to highly sophisticated uses of satellite imagery. Although most G20 countries publish quasi-regularly annual wildfire statistics in their official languages, data on individual fires is generally scarce. To make matters worse, there is hardly any consistency in either content or the granularity of the information reported across countries.
Finally, although the short- and long-term effects of wildfires on forests vary with the ability of tree species to regenerate after fires, both the size and costs of wildland fire suppression operations are growing. The increase in cost is partially driven by the high cost of protecting property in the WUI, and urbanization will drive costs even higher. Wildfire suppression costs in the United States have increased fourfold from 1985 to 2016 ( 21 ).
Wading into uncharted waters, governments have mobilized funding for improving suppression capabilities, with aerial firefighting absorbing the lion's share. Improvements in preparedness tend to follow particularly destructive fires or very active fire seasons. For example, following an increase in wildfire incidence across EU countries, the European Commission established “rescEU”, for procuring fixed and rotary wing firefighting aircraft, to create a last-resort capability buffer. Thus, rescEU's aerial firefighting fleet was doubled in 2023, reaching 28 aircraft in 2023, with 12 additional aircraft on order ( 66 , 67 ).
Greece has relied on a combined aerial firefighting fleet of nationally owned and leased aircraft. The country found itself in a double predicament, facing increasingly devastating fire seasons, just as it was getting out of a deep financial crisis, which had essentially prevented the acquisition of expensive assets. It has been progressively leasing more firefighting helicopters and airplanes. Following a series of devastating fire seasons, in 2020, the government announced “Aegis”, a behemoth two-billion-euro program, a third of which will serve to essentially double the country's aerial fighting fleet. In 2022, the Hellenic Fire Service also instituted helitack crews, that is, teams of firefighters transported by helicopter to poorly accessible wildfire flare ups.
France faced a particularly active season in 2022. The total burnt area was 72,000 hectares, or almost five times that of 2021 and about three times that of 2017. Preparing for 2023, it added nine additional fixed and rotary wing aircraft to its 38. It also increased the number of wildland fire-engine strike teams from 44 in 2022 to 51 in 2023 ( 68 ). According to the US Congressional Budget Office ( 69 ), the federal government spent $17.5 billion on wildfire suppression and disaster assistance between 2016 and 2020. In California, following expanding fire seasons and a series of devastating fires, the Department of Forestry and Fire Protection recently acquired 12 new firefighting helicopters and is planning to add seven air tankers to its fleet ( 70 ).
On the other hand, wildfire mitigation and prevention have traditionally received less attention and funding. For instance, the Greek Forest Service had been understaffed, and activities poorly coordinated and underfunded, for decades ( 71 ). Feo et al. ( 72 ) noted a “long history of underinvestment in prevention and mitigation” in California. These are examples of how voters often reward politicians for delivering disaster relief, but not for investing in preparedness, and even less so for mitigation and adaptation, hence governments underinvest ( 73 ).
Moritz et al. ( 19 ) have argued that “cultural and institutional systems affect public response to wildfire, as do psychological and social dynamics”. While true overall, the death toll in recent WUI fires suggests the public is inching towards favoring evacuation. Recent research suggests that public education can motivate voter support in favor of mitigation and preparedness ( 74 ). Anecdotal wildfire policy examples suggest that climate change education in the last few years may have helped to sway public opinion in favor of wildfire mitigation. For instance, in preparation for the 2022 fire season, the Greek government spent 72 million euros, equivalent to the cost of two medium-sized firefighting airplanes, in two nationwide wildfire hazard mitigation programs, involving (i) fuel reduction and clearing and (ii) forest thinning; such an investment was unthinkable five years earlier. It also reorganized the Forest Service to improve coordination and funding. In 2020 and 2021, the State of California appropriated $2.7 billion over a four-year period to wildfire prevention and mitigation, a number which dwarfs the Federal Government's $4 billion through the Infrastructure Investment and Jobs Act and the Inflation Reduction Act ( 75 ).
With rising fire activity brought on by climate change and human development, and increased exposure of people and property in WUI areas, it is unlikely that today's suppression-focused paradigm will remain sustainable, possibly not even in the near future. Here, we present a new paradigm in wildfire risk management that goes beyond suppression and even mitigation, by integrating science-based land management.
First, in light of the projected increase in fire activity and decades of unmitigated biomass accumulation in forests around the world, maintaining adequate fire suppression capabilities, even through mutual aid agreements, may no longer be a fiscally responsible option. On the other hand, the cost-to-benefit ratios of wildfire hazard mitigation measures, including retrofitting buildings to meet or exceed building code standards, range from ¼ to ½ ( 76 ). Communities should consider mitigation strategies that have worked for other hazards, such as insurance and drastically limiting development in the WUI. Fuel treatment strategies, such as prescribed burning, fuel reduction and clearing, and forest thinning, have all shown potential in mitigating wildland fire risk ( 2 , 77 ). In addition, retrofitting properties in the WUI to resist fire hazards has paid off time and again ( 78 ). For instance, a photograph of a 100-year-old wooden house in Lahaina became viral in the aftermath of the August 8 fire, as it was left unscathed while surrounded by piles of charred debris (Figure 3 ). It was later revealed that the owners had retrofitted the home to mitigate wildfire risk. Furthermore, learning from indigenous fire stewardship practices can help mitigate wildfires and increase biodiversity ( 79 ).

Red-roofed Lahaina home left unscathed by the August 8 fire (Source: Getty Images).
Nevertheless, even with increased investment, implementing hazard mitigation strategies and developing additional capabilities will take time. Therefore, improving wildfire response is still needed. Given resource constraints, communities will likely have to prioritize the development of both suppression and mitigation capabilities by accounting for the effects of climate change on forest ecosystems. Multi-year, integrated wildfire risk management plans extending beyond electoral cycles can help reduce the sensitivity of risk management to shifting political short-term priorities and ensure sustainable strategies. Governments need to avoid the established pitfalls of short-sighted, political decision-making and policy choices shaped by what just happened instead of planning for future expectations ( 80 ).
Second, the growing number of megafires indicates that land management strategies need to account for the impact of climate change on forest ecosystems, as well as local effects, such as the rate at which biomass re-accumulates. Governments need to work closer with the forest products industry to integrate land management and wildfire risk management policies. Heterogeneity in vegetation type, age, and structure can help ecosystem resilience to climate change and reduction of biodiversity loss. Fire hazard mitigation measures can be adapted to deliver the needed heterogeneity, for example, by diversifying the conditions of prescribed burns ( 19 ). Moreover, novel uses of wood products and creating markets for excess biomass and smallwood removal and utilization can create local economic development opportunities, while increasing the ecosystem's resilience to wildfires. Several funding mechanisms are now being considered to support appropriate public/private partnerships. Examples include green bonds and more complex schemes, such as partial subsidization of economic opportunities, contingent on the implementation of wildfire mitigation or post-wildfire restoration measures, including landslide and flood mitigation.
Third, in terms of crisis management, a fundamental shift is needed from a mindset of fire suppression to one of emergency response. The increased exposure of people reinforces the need for community-based evacuation planning. Evacuation plans identify transportation routes and ways to increase evacuation throughput (such as contraflow), transportation modes, including mass transit, and shelter locations, so that emergency managers know how little time they have to issue evacuation orders. It is thus clear that public alert and warning systems are becoming an increasingly critical link in the process. These systems provide emergency information to the public, and save lives when disasters are about to strike. Early disaster research ( 81 , 82 ) highlighted that to be effective warning messages need to be received from multiple communication pathways and issued from credible sources. Modern public alert and warning systems enable emergency management agencies to disseminate information through multiple pathways, including mobile and landline phones, radio, television, highway variable-message signs and others. The combination of evacuation plans, public education before disaster strikes, and public alert and warning systems can create a powerful defense against wildland fires and can reduce the loss of life.
Because of the inherent complexity in combining all those technologies, these systems should be tested to the limit of their abilities at regular intervals. In addition to the obvious maintenance benefit, these tests are opportunities for informing the public about the capabilities of alert and warning systems, as well teaching individual and family self-protection in disasters. In the United States, nationwide tests of the Emergency Alert System (which is based on radio and television, cable systems, and satellite radio and television) and the Wireless Emergency Alert (WEA) system, (which delivers alerts from cellular towers to mobile devices using a one-to-many technology called Cell Broadcast (CB)), they have been required no less than every three years since 2015. Nonetheless, the third nationwide test on October 4, 2023 (Figure 4 , left), which came after the Maui catastrophe ( 83 ), was treated by the major news media everywhere as if it was the first ever. In one way it was, because people paid attention to it and could not have opted out, as was the case with the second test message. For the record, the first test was conducted on October 3, 2018, using the Presidential Alert classification and the second on August 11, 2021, using the State/Local WEA Test classification, which requires users to opt in to receive the test alert.

Screenshots of the 2023 nationwide WEA test in the United States (left) and the first nationwide Cell Broadcast message ever in Greece, in 2020 (right). An estimated 300 national messages have followed since then this 11 March 2020 first CB message.
As of December 2018, and likely following the realization that lives could have been saved in the Mati fires earlier that year had there been warnings, European Union countries have been legally required to put in place public alert and warning systems using location-based SMS, or similar technologies, no later than June 2022. However, there are no testing requirements. Although such systems were already used in several European countries before that deadline, Greece was one of the first countries to use Cell Broadcast for wildfire evacuations. Tests are not legally required in Greece as in the United States, but its first nationwide alert was sent on March 11, 2020 (Figure 4 , right), in response to mounting cases of COVID-19, and served as a demonstration of the capability.
Warning and evacuation processes also need to account for populations with access and functional needs. Effective systems can alert populations with disabilities or other special needs, include those with limited proficiency in the main language(s) used in alert and warning messages and, ultimately, anyone who may have difficulties obtaining alert and warning SMS and information.
A combination of forward and reverse planning is required to prepare such plans ( 84 , 85 ). Forward planning builds the evacuation process by describing potential decisions and actions sequentially. Plans should address both agent-generated demands (for example, transportation and shelter) and response-generated demands (for example, information management and logistics). Then, reverse planning determines the time required to complete each task, given the resources that can realistically be made available. Working backwards and using time estimates and task dependencies, emergency managers can identify the times required to complete evacuations once the decision is made. The combination of forward and reverse planning informs the development of time or land-benchmark triggers for evacuation decision-making. For example, there will be evacuations regardless of conditions, if a fire reaches a particular critical location, or if there is less than x minutes for the fire to spread from its point of ignition to the nearest shoreline.
Fundamentally, hard as it may be in practice, evacuations need to be completed before the flame front spreads close enough to populated areas. Fire smoke may cause serious injury to sensitive individuals; this needs to be accounted for as well. Evacuation modeling is necessary to inform evacuation planning and decision-making. It can provide estimates of the time needed to evacuate areas threatened by wildfires, as well as pedestrian and vehicle traffic flow rates, in different scenarios and allows analysis of different courses of action ( 86 , 87 ). As was done post-facto in Mati ( 88 , 89 ), combined with wildfire spread simulation, evacuation modeling can be a powerful tool in setting triggers for evacuation decision-making ( 90 ). Stefanakis et al. ( 91 ) used an AI statistical model of a tsunami “experiment” constantly updated as new results arrived, then future query points were selected according to the objectives, until achieved. In this manner, they identified worst-case scenarios that were previously only obvious with 100% hindsight. Such active experimental design and machine learning algorithms can reduce the exceptionally large number of numerical simulations needed to identify time-optimal escape routes in advance, particularly in the presence of projectiles (embers), which change the spread dynamics. Evacuation modeling is also a powerful tool in public outreach, as people at risk can expect how much time they really have. Coupled with avatars, people can visualize themselves evacuating, at different speeds, temperatures, and visibilities. Such an approach could become the gold standard for planning evacuations and educating people.
Fourth, science and technology can be leveraged to improve strategic, operational, and tactical emergency planning. Evaluating variables, such as meteorological conditions, fire danger, fire location, time of day, and fire size at the time of dispatch are now increasingly used in setting dispatch levels and resource estimates, along with lessons learned from previous fires and institutional memory. Machine learning, again, can streamline these processes. For example, Lam et al. ( 92 ) show how it can best medium-range numerical weather predictions (NWP) forecasts very quickly. Introducing AI fire prediction operationally remains vexing, given the varied locations, diverse data, and one-of-a-kind fires, even in a single locale.
Even when such timely predictions materialize, resource management should become more dynamic and anticipatory through the employment of other tools. For instance, mutual aid agreements can be activated preemptively based on risk estimates. In 2023, Greece activated the EU Civil Protection Mechanism (the European equivalent to the Emergency Management Assistance Compact in the United States) to preposition fire engines and hand crews from other countries in high-risk areas, during the hottest and driest summer months. The Hellenic Fire Service hires seasonal firefighters every year, aiming to increase its force by 10% during the “hot” season ( 8 ). These actions are particularly useful in islands, which present additional tactical challenges, not the least of which is related to their insularity and limited options for evacuation. Emergency management worldwide needs to consider the option of evacuating to beaches, which has made fires on Greek islands far less deadly than the mainland.
Earlier, we discussed how advances in forestry and biology can support integrated management of wildfire risk, how cutting-edge communications systems enable alert and warning messages to reach millions of people in seconds, and how evacuation modeling can support emergency planning and rapid decision-making.
Satellite images are a staple in many crisis management centers. Landsat has been around for decades, but the time windows between images do not allow for effective proactive modeling. Algorithms convert observations of the Earth's surface in the visible and infrared domains from meteorological and other satellites into measurements of fire hot spots and assessments of wildfire impacts in near real-time ( 93–95 ). Recently, advances in earth observation have allowed scientists, at least in Europe and North America, to track wildfires and even calculate fire spread, every twelve hours, based on satellite imagery ( 96 ). NASA's Fire Events Data Suite is claimed to provide more frequent monitoring of fire activity, growth, and behavior than has ever been available ( 59 ). In a significant breakthrough, private operating satellite constellations now claim they are able to provide end users with predictions of the spread of wildfires and floods, informed through data assimilation, as images become available from successive satellite passes. In one example, a satellite constellation of Portugal and Spain, possibly to be augmented by Greek satellites, beginning (it's been claimed) in 2024, will provide high resolution synthetic aperture radar (SAR) images about every four hours. The consortium will also provide estimates of the projected evolution of the fire perimeter, until the next set of images becomes available. When augmented by thermal imaging and comprehensive vegetation spatial distributions, the evolution predictions can improve further and can become game changers in megafire suppression. Once such analyses become standard, AI fire modeling could become routine, given that there will be uniform image datasets associated with specific weather parameters to mine from.
Moreover, artificial intelligence is already in use to streamline real-time or near real-time detection of wildfires in poorly accessible areas ( 97 , 98 ). State-of-the-art robots provide situational awareness, and autonomous systems operating closer to the fireline are under development for improving information management ( 99 ). The integration of satellite imagery with feeds from other sources, such as thermal, surveillance and traffic cameras and weather stations, they can vastly accelerate information management in the Emergency Operations Centers of the next decade.
Climate change, increased development in the WUI, and a long history of emphasis on fire suppression over hazard mitigation has increased wildfire risk worldwide. Governments are exploring the issue, but spending is mostly geared toward fire suppression. Here, we propose a long-term approach, incorporating wildfire risk management in wider-scope forest land management policies and strategies. The latter need to account for the impact of climate change and hazard mitigation on forest ecosystems. Improving suppression capabilities is like performing CPR on a cardiac arrest patient: it buys needed time until a more sustainable strategy takes effect, but will not, in and by itself, suffice for longevity. A renewed focus and increased spending on hazard mitigation and fire prevention is also required to stem the impact of megafires threatening human settlements and ecosystems in the WUI. Furthermore, a fundamental shift is needed from a mindset of fire suppression to one of emergency response. Evacuation planning supported by public alert and warning capabilities always saves lives, and more so with prior planning. Last, we urge the wildland fire community to invest in the uptake of scientific breakthroughs and new technologies, including machine learning and earth observation, to help improve decision-making and, ultimately, save more lives. Until then, in at-risk WUI areas, everyone needs to understand that a threatening wildfire could happen anytime from spring to fall and that they may need to leave immediately.
The authors declare no funding.
Both authors designed and performed research, analyzed the data, and wrote the paper.
The following data were used in the preparation of this manuscript:
[dataset] CRED/UCLouvain, n.d., “EM-DAT”. Brussels, Belgium: University of Louvain. www.emdat.be .
[dataset] European Forest Fire Information System (EFFIS), n.d., “Burnt Areas database”. European Commission. [accessed 2023 Nov 12]. https://forest-fire.emergency.copernicus.eu/apps/effis.statistics/estimates .
[dataset] Natural Resources Canada (NRC), n.d., “National Fire Database fire point data”. [accessed 2023 Nov 12]. https://cwfis.cfs.nrcan.gc.ca/datamart/download/nfdbpnt .
[dataset] National Interagency Fire Center (NIFC), n.d., “Wildland Fire Incident Locations”. [accessed 2023 Nov 12]. https://data-nifc.opendata.arcgis.com/datasets/nifc::wildland-fire-incident-locations/explore .
All of the data listed above are publicly available to download at no charge by the respective organizations.
Lu M , Ikejiri T , Lu Y-H . 2021 . A synthesis of the Devonian wildfire record: implications for paleogeography, fossil flora, and paleoclimate . Palaeogeo Palaeoclimat Palaeoeco 571 ( 1 ): 110321 . https://doi.org/10.1016/j.palaeo.2021.110321
Google Scholar
Bowman DMJS , et al. 2011 . The human dimension of fire regimes on earth . J Biogeogr . 38 : 2223 – 2236 .
van Wagtendonk JW . 2007 . The history and evolution of wildland fire use . Fire Ecol . 3 : 3 – 17 .
Associated Press . 2024. The death toll from Chile's wildfires reaches 131, and more than 300 people are missing. [accessed 2024 Feb]. https://apnews.com/article/chile-deadly-wildfires-valparaiso-d40a40ed31d14d7901080f0d700d2afa .
Akbarzai S , Paddison L . 2024. Wildfires that are turning neighborhoods to ash are likely Chile's deadliest on record, UN agency says. CNN. [accessed 2024 Feb]. https://edition.cnn.com/2024/02/06/climate/chile-wildfires-deadliest-climate-intl/index.html .
National Fire Protection Association (NFPA) . 2023. Wildland Fires in US History with Ten or More Fatalities. Available at: https://www.nfpa.org/-/media/Files/News-and-Research/Fire-statistics-and-reports/WUI/Wildland-Fires-in-the-US-history-with-10-or-more-deaths.ashx (August 2023).
Synolakis CE . 2023. The Fire in Maui and the Lesson of Greece. Wall Street Journal. Available at: https://www.wsj.com/articles/lahaina-and-the-fires-in-greece-firefighters-natural-disaster-evacuations-maui-emergency-hawaii-fa99f1c0 (August 13, 2023).
Synolakis CE , Karagiannis GM . 2023. Why Didn’t Hawaii Evacuate Sooner During the Fires?. The New York Times. Available at: https://www.nytimes.com/2023/08/19/opinion/maui-wildfires-hawaii-sirens.html (August 19, 2023).
National Academies of Sciences, Engineering, and Medicine (NASEM) . 2022 . The chemistry of fires at the wildland-urban interface . Washington (DC) : The National Academies Press .
Google Preview
Kollanus V , et al. 2017 . Mortality due to vegetation fire-originated PM 2.5 exposure in Europe—assessment for the years 2005 and 2008 . Environ Health Perspect . 125 ( 1 ): 30 – 37 .
Rooney B , et al. 2020 . Air quality impact of the Northern California camp fire of November 2018 . Atm Chem Phys . 20 ( 23 ): 14597 – 14616 . https://doi.org/10.5194/acp-20-14597-2020
Chen G , et al. 2021 . Mortality risk attributable to wildfire-related PM 2.5 pollution: a global time series study in 749 locations . Lancet Plan Health . 5 ( 9 ): E579 – E587 .
Okal EA , Plafker G , Synolakis CE , Borrero JC . 2003 . Near-field survey of the 1946 Aleutian tsunami on Unimak and Sanak Islands . Seism Soc Am Bul . 93 : 1226 – 1234 .
McEntire DA . 2022 . Disaster response and recovery: strategies and tactics for resilience . Third Edition. Hoboken (NJ) : Wiley .
Burgan RE , Fujioka FM , Hirata GH . 1974 . A fire danger rating system for Hawaii . Fire Technol . 10 : 275 – 281 .
Kantouris C , Gatopoulos D . 2018. Greek fire damages over 2,000 homes, 500 of them gutted. Associated Press. Available at: https://apnews.com/article/fires-forensics-greece-wildfires-international-news-d364517576614fc78022d1993a491aed (July 27, 2018).
BBC . 2023. Greece fires in maps and satellite images show extent of damage. Available at: https://www.bbc.com/news/world-europe-66295972 (July 27, 2023).
Nellas D . 2023. 2,000 people including tourists evacuated as a wildfire rages on the Greek island of Rhodes. Associated Press. Available at: https://apnews.com/article/fire-rhodes-greece-evacuations-heat-wave-1a9fb0d687ce22c9087bf83ac7973cf1 (July 22, 2023).
Moritz MA , et al. 2014 . Learning to coexist with wildfire . Nature . 515 : 58 – 66 .
Krawchuck MA , Moritz MA . 2011 . Constraints on global fire activity vary across a resource gradient . Ecology . 92 ( 1 ): 121 – 132 .
USGCRP . 2018 . [ Reidmiller DR , et al. (eds.)], Impacts, risks, and adaptation in the United States: fourth national climate assessment , Volume II . Washington, DC, USA : US Global Change Research Program .
Ganteaume A , et al. 2013 . A review of the main driving factors of forest fire ignition over Europe . Environ Manage . 51 ( 3 ): 651 – 662 .
Radeloff VC , et al. 2023 . Rising wildfire risk to houses in the United States especially in grasslands and scrublands . Science . 382 : 702 – 707 .
CRED/UCLouvain . 2023. [dataset] n.d ., EM-DAT . Brussels, Belgium : University of Louvain .
Gorte RW , Bracmort K . 2012 . Wildfire protection in the wildland urban interface . Washington (DC) : Congressional Research Service .
National Wildfire Coordinating Group (NWCG) . 2014. Wildland fire incident management field guide . https://gacc.nifc.gov/swcc/dc/nmadc/dispatch_logistics/dispatch/documents/2014%20Wildland%20Fire%20Incident%20Management%20Field%20Guide.pdf .
Lampin-Maillet C , et al. 2010 . Mapping wildland-urban interfaces at large scales integrating housing density and vegetation aggregation for fire prevention in the South of France . J Environ Manage . 91 ( 3 ): 732 – 741 .
United States Fire Administration (USFA) . 2002 . Fires in the wildland/urban interface . US Fire Admin Top Fire Res Ser . 2 ( 16) . https://apps.usfa.fema.gov/downloads/pdf/statistics/v2i16-508.pdf .
McLennan J , Ryan B , Bearman C , Toh K . 2019 . Should we leave now? Behavioral factors in evacuation under wildfire threat . Fire Technol . 55 : 487 – 516 .
Benali A , Aparicio BA , Goncalves A , Oliveira S . 2023 . Defining priorities for wildfire mitigation actions at the local scale: insights from a novel risk analysis method applied in Portugal . Front For Glob Change . 6 : 1270210 . https://doi.org/10.3389/ffgc.2023.1270210
United States Fire Administration (USFA) . 2022. Wildland Urban Interface: A Look at Issues and Resolutions. US Fire Administration. [accessed 2024 Apr 27]. https://www.usfa.fema.gov/downloads/pdf/publications/wui-issues-resolutions-report.pdf .
Synolakis CE . 2007. Olympic Torching. Wall Street Journal. Available at: https://www.wsj.com/articles/SB118816586120409172 (August 27, 2007).
Xanthopoulos G , Viegas DX , Caballero D . 2009. The fatal fire entrapment accident of August 24, 2007, near the village of Artemida, Ilia, Greece. Abstracts from the 10 th Wildland Fire Summit, Phoenix, Arizona. [accessed 2024 May 6]. https://www.researchgate.net/profile/Gavriil-Xanthopoulos-2/publication/285541773_The_fatal_fire_entrapment_of_Artemida_Greece_2007/links/56653a2f08ae15e746330760/The-fatal-fire-entrapment-of-Artemida-Greece-2007.pdf .
Szeto W . 2022. Bracing for wildfire season, residents of this West Kelowna neighbourhood are asking for more exit routes. CBC. Available at: https://www.cbc.ca/news/canada/british-columbia/west-kelowna-wildfire-glenrosa-road-traffic-congestion-1.6412905 (April 2022).
Synolakis C . 2018. Lessons from the Paradise Fire. The Wall Street Journal. Available at: https://www.wsj.com/articles/lessons-from-the-paradise-fire-1542577702 (November 18, 2018).
McLennan J , Holgate AM , Omodei MM , Wearing AJ . 2006 . Decision making effectiveness in wildfire incident management teams . J Conting Crisis Manag . 14 ( 1 ): 27 – 37 .
Hand M , Katuwal H , Calkin DE , Thompson MP . 2017 . The influence of incident management teams on the deployment of wildfire suppression resources . Int J Wild Fire . 26 ( 7 ): 615 – 629 .
Thompson MP . 2013 . Modeling wildfire incident complexity dynamics . PLoS One . 8 ( 5 ): e63297 . https://doi.org/10.1371/journal.pone.0063297
Thomspon MP . 2014 . Social, institutional, and psychological factors affecting wildfire incident decision making . Soc Nat Resourc . 27 ( 6 ): 636 – 644 .
Pescaroli G , Alexander D . 2018 . Understanding compound, interconnected, interacting, and cascading risks: a holistic framework . Risk Anal . 38 ( 11 ): 2245 – 2257 .
Pescaroli G , et al. 2023 . Managing systemic risk in emergency management, organizational resilience and climate change adaptation . Disast Prev Manag . 32 ( 1 ): 234 – 251 .
Mitchell JW . 2013 . Power line failures and catastrophic wildfires under extreme weather conditions . Eng Fail Anal . 35 : 726 – 735 .
Alexander D . 2000 . Confronting catastrophe: new perspectives on natural disasters . Hertfordshire, UK : Terra Publishing .
Kern H , Krausmann E . 2020 . Wildfires triggering natech events . Luxembourg : Publications Office of the European Union .
Bowman DMJS , et al. 2009 . Fire in the earth system . Science . 324 ( 5926 ): 481 – 484 .
Flannigan M , et al. 2013 . Global wildland fire season severity in the 21st century . For Ecol Manag . 294 : 54 – 61 . https://doi.org/10.1016/j.foreco.2012.10.022
Intergovernmental Panel on Climate Change (IPCC) . 2022 . Climate change 2022: impacts, adaptation and vulnerability. Contribution of working group II to the sixth assessment report of the intergovernmental panel on climate change . Cambridge, UK and New York, NY, USA : Cambridge University Press .
Zhuang Y , Fu R , Santer BD , Dickinson RE , Hall A . 2021 . Quantifying contributions of natural variability and anthropogenic forcings on increased fire weather risk over the western United States . Proc Natl Acad Sci U S A . 118 ( 45 ):e2111875118. https://doi.org/10.1073/pnas.2111875118
Barbero R , Abatzoglou JT , Larkin NK , Kolden CA , Stocks B . 2015 . Climate change presents increased potential for very large fires in the contiguous United States . Int J Wild Fire . 24 : 892 – 899 .
Balch JK , et al. 2022 . Warming weakens the night-time barrier to global fire . Nature . 602 : 442 – 448 . https://doi.org/10.1038/s41586-021-04325-1
Yue X , Mickley LJ , Logan JA . 2014 . Projection of wildfire activity in southern California in the mid-twenty-first century . Clim Dyn . 43 : 1973 – 1991 .
Litschert SE , Brown TC , Theobald DM . 2012 . Historic and future extent of wildfires in the Southern Rockies Ecoregion, USA . For Ecol & Man . 269 : 124 – 133 .
Prestemon JP , et al. 2016 . Projecting wildfire area burned in the south-eastern United States, 2011–60 . Int J Wild Fire . 25 : 715 – 729 .
San Miguel J , et al. 2017 . Climatological risk: wildfires. In: Poljanšek K , Marín Ferrer M , De Groeve T , Clark I , editors. Science for disaster risk management 2017: knowing better and losing less . Luxembourg : Publications Office of the European Union .
Dowdy AJ , et al. 2019 . Future changes in extreme weather and pyroconvection risk factors for Australian wildfires . Sci Rep . 9 : 10073 .
Costa H , de Rigo D , Libertà G , Houston Durrant T , San-Miguel-Ayanz J . 2020 . European Wildfire danger and vulnerability in a changing climate: towards integrating risk dimensions . Luxembourg : Publications Office of the European Union .
Belcher CM , et al. 2021 . UK Wildfires and their climate challenges . Exeter, UK : University of Exeter Global Systems Institute .
Horton H . 2022. Summer wildfires increased fourfold in England in 2022. The Guardian. Available at: https://www.theguardian.com/world/2022/dec/30/summer-wildfires-increased-fourfold-in-england-in-2022 (December 30, 2022).
National Aeronautical and Space Agency (NASA) . 2023. Tracking Canada's Extreme 2023 Fire Season. Available at: https://earthobservatory.nasa.gov/images/151985/tracking-canadas-extreme-2023-fire-season (July 23, 2023).
Abatzoglou JT , Cohen CA . 2013 . Relationships between climate and macroscale area burned in the western United States . Int J Wild Fire . 22 : 1003 – 1020 .
Donovan VM , Wonkka CL , Twidwell D . 2017 . Surging wildfire activity in a grassland biome . Geoph Res Let . 44 : 5986 – 5993 .
Luo K , Wang X , de Jong M , Flannigan M . 2024 . Drought triggers and sustains overnight fires in North America . Nature . 627 : 321 – 327 . https://doi.org/10.1038/s41586-024-07028-5
[dataset] European Forest Fire Information System (EFFIS). n.d., Burnt Areas database. European Commission. [accessed 2023 Nov 12]. https://forest-fire.emergency.copernicus.eu/apps/effis.statistics/estimates .
[dataset] Natural Resources Canada (NRC), n.d . National Fire Database fire point data. Available at https://cwfis.cfs.nrcan.gc.ca/datamart/download/nfdbpnt (accessed November 12, 2023).
[dataset] National Interagency Fire Center (NIFC) , n.d. Wildland Fire Incident Locations. Available at: https://data-nifc.opendata.arcgis.com/datasets/nifc::wildland-fire-incident-locations/explore (accessed November 12, 2023).
Abnett K . 2023. EU plans to buy new firefighting planes as climate crises worsen, Reuters. Available at: https://www.reuters.com/world/europe/eu-plans-buy-new-firefighting-planes-climate-crises-worsen-2023-07-26/ (July 26, 2023).
Tennant C . 2023. Will the EU's €720 million gamble on firefighting planes pay off?, Euronews. Available at: https://www.euronews.com/my-europe/2023/09/17/will-the-eus-720-million-gamble-on-firefighting-planes-pay-off (September 17, 2023).
Ministère de l’Intérieur et des Outre-mer (MIOM), Ministère de la Transition écologique et de la Cohésion des territoires, Ministère de l’Agriculture et de la Souveraineté alimentaire, “Lutte contre les feux de forêt: protéger les populations, les biens et l’environnement—Dossier de presse 2023”. Available at: https://agriculture.gouv.fr/telecharger/136293 (April 11, 2023).
US Congressional Budget Office (CBO) . 2022. Wildfires. [accessed 2022 Jun]. https://www.cbo.gov/system/files/2022-06/57970-Wildfires.pdf .
California Department of Forestry and Fire Protection (CALFIRE), Aviation Program. Available at: https://www.fire.ca.gov/what-we-do/fire-protection/aviation-program (Accessed October 2023).
Pandey P , et al. 2023 . A global outlook on increasing wildfire risk: current policy situation and future pathways . Tr For Peo . 14 : 100431 .
Feo TJ , Mace AJ , Brady SE , Lindsey B . 2020 . The costs of wildfire in California—an independent review of scientific and technical information . Sacramento (CA) : California Council on Science and Technology .
Healy A , Malhotra N . 2009 . Myopic voters and natural disaster policy . Am Pol Sci Rev . 103 ( 3 ): 387 – 406 .
Anderson SE , DeLeo R , Taylor K . 2023 . Legislators do not harness voter support for disaster preparedness . Risk Haz Cris in Pub Pol . 14 ( 1 ): 68 – 88 .
Foard C , et al. 2022. Wildfires: Burning Through State Budgets. The Pew Charitable Trusts. Available at: https://www.pewtrusts.org/-/media/assets/2022/11/wildfires-burning-through-state-budgets.pdf (November 2022).
Porter K , et al. 2019. Natural hazard mitigation saves: 2019 report . Washington (DC) : National Institute of Building Sciences .
United States Forest Service (USFS) . Confronting the Wildfire Crisis: A Strategy for Protecting Communities and Improving Resilience in America's Forests. Available at: https://www.fs.usda.gov/sites/default/files/fs_media/fs_document/Confronting-the-Wildfire-Crisis.pdf (January 2022).
Penman TD , et al. 2017 . Retrofitting for wildfire resilience: what is the cost? Int J Dis Risk Red . 21 : 1 – 10 .
Hoffman KM , et al. 2021 . Conservation of Earth's biodiversity is embedded in indigenous fire stewardship . Proc Natl Acad Sci U S A . 118 ( 32 ):e2105073118. https://doi.org/10.1073/pnas.2105073118
Mulligan TD , Taylor K , DeLeo RA . 2019 . Politics and policies for managing natural hazards . Oxf Res Enc on Nat Haz Sci . https://doi.org/10.1093/acrefore/9780199389407.013.314
Mileti DS , Sorensen J . 1990 . Communication of emergency public warning: A social science perspective and state-of-the-art assessment . Oak Ridge (TN) : Oak Ridge National Laboratory .
Quarantelli EL . 1990 . The warning process and evacuation behavior: the research evidence . Newark (DE) : Disaster Research Center, University of Delaware .
Carballo R . 2023. Nationwide Alert Reaches Cellphones, TVs and Radios. The New York Times. Available at: https://www.nytimes.com/2023/08/30/us/test-alert-us-october.html (October 4, 2023).
Karagiannis GM , Synolakis CE . 2017 . Twenty challenges in incident planning . J Hom Sec Emer Man . 14 ( 2 ): 20160061 . https://doi.org/10.1515/jhsem-2016-0061
Federal Emergency Management Agency (FEMA) . 2010 . Developing and maintaining emergency operations plans—comprehensive preparedness guide (CPG) 101—version 2.0 . Washington (DC) : US Department of Homeland Security .
Zhao B , Wong SD . 2021 . Developing transportation response strategies for wildfire evacuations via an empirically supported traffic simulation of Berkeley, California . Trans Res Rec . 2675 ( 12 ): 557 – 582 .
Pishahang M , Ruiz-Tagle A , Lopez Droguett E , Ramos M , Mosleh A . 2024. WISE: A Probabilistic Wildfire Safe Egress Planning Framework and Software Platform. Probabilistic Safety Assessment and Management PSAM 16, Honolulu, HI. Available at: https://www.iapsam.org/PSAM16/papers/ALL-PSAM16-PAPERS.zip (April 3, 2024).
Synolakis C . 2018. The deadly fire of July 23rd at Mati: Fire and evacuation modeling [Greek]. Available at: https://www.youtube.com/watch?v=CTPUEyNh7-0 (September 2018).
Synolakis C , Karagiannis G . 2023. Heatwaves and wildfires: How Greece is handling the double whammy. eKathimerini. Available at: https://www.ekathimerini.com/opinion/1216624/heatwaves-and-wildfires-how-greece-is-handling-the-double-whammy/ (July 30, 2023).
Li D , Cova TJ , Dennison PE . 2018 . Setting wildfire evacuation triggers by coupling fire and traffic simulation models: a spatiotemporal GIS approach . Fire Tech . 55 : 617 – 642 https://doi.org/10.1007/s10694-018-0771-6
Stefanakis TS , Contal E , Vayatis N , Dias F , Synolakis CE . 2014 . Can small islands protect nearby coasts from tsunamis? An active experimental design approach . Proc R Soc A . 470 : 20140575 . https://doi.org/10.1098/rspa.2014.0575
Lam R , et al. 2023 . Learning skillful medium-range global weather forecasting . Science . 382 : 1416 – 1421 https://doi.org/10.1126/science.adi2336
Jiao L , Bo Y . 2022 . Near real-time mapping of burned area by synergizing multiple satellites remote-sensing data . GISci Rem Sens . 59 ( 1 ): 1956 – 1977 . https://doi.org/10.1080/15481603.2022.2143690
Thangavel K , Spiller D , Sabatini R , Marzocca P , Esposito M . 2023 . Near real-time wildfire management using distributed satellite system . IEEE Geosci Rem Sens Lett . 20 : 5500705 . https://doi.org/10.1109/LGRS.2022.3229173
Andela N , et al. 2022 . Tracking and classifying Amazon fire events in near real time . Sci Adv . 8 ( 30 ): eabd2713 . https://doi.org/10.1126/sciadv.abd2713
Chen Y , et al. 2022 . California wildfire spread derived using VIIRS satellite observations and an object-based tracking system . Sci Rep . 9 : 249 .
Fuller T . 2023. Can AI detect wildfires faster than humans? California is trying to find out. The New York Times. Available at: https://www.nytimes.com/2023/08/24/us/wildfires-ai-detection-california.html (August 24, 2023).
Ban Y , Zhang P , Nascetti A , Bevington AR , Wulder MA . 2020 . Near real-time wildfire progression monitoring with sentinel-1 SAR time series and deep learning . Sci Rep . 10 : 1322 . https://doi.org/10.1038/s41598-019-56967-x
Colajanni G , Daniele P , Nagurney A , Nagurney L , Sciacca D . 2023 . A three-stage stochastic optimization model integrating 5G technology and UAVs for disaster management . J Glob Optimiz . 86 : 741 – 780 . doi: 0.1007/s10898-023-01274-z
Author notes
Email alerts, citing articles via.
- Contact PNAS Nexus
- Advertising and Corporate Services
- Journals Career Network
Affiliations
- Online ISSN 2752-6542
- Copyright © 2024 National Academy of Sciences
- About Oxford Academic
- Publish journals with us
- University press partners
- What we publish
- New features
- Open access
- Institutional account management
- Rights and permissions
- Get help with access
- Accessibility
- Advertising
- Media enquiries
- Oxford University Press
- Oxford Languages
- University of Oxford
Oxford University Press is a department of the University of Oxford. It furthers the University's objective of excellence in research, scholarship, and education by publishing worldwide
- Copyright © 2024 Oxford University Press
- Cookie settings
- Cookie policy
- Privacy policy
- Legal notice
This Feature Is Available To Subscribers Only
Sign In or Create an Account
This PDF is available to Subscribers Only
For full access to this pdf, sign in to an existing account, or purchase an annual subscription.
Thank you for visiting nature.com. You are using a browser version with limited support for CSS. To obtain the best experience, we recommend you use a more up to date browser (or turn off compatibility mode in Internet Explorer). In the meantime, to ensure continued support, we are displaying the site without styles and JavaScript.
- View all journals
- My Account Login
- Explore content
- About the journal
- Publish with us
- Sign up for alerts
- Open access
- Published: 05 September 2023
Abrupt, climate-induced increase in wildfires in British Columbia since the mid-2000s
- Marc-André Parisien ORCID: orcid.org/0000-0002-8158-7434 1 ,
- Quinn E. Barber 1 ,
- Mathieu L. Bourbonnais 2 ,
- Lori D. Daniels 3 ,
- Mike D. Flannigan 4 ,
- Robert W. Gray 5 ,
- Kira M. Hoffman 3 ,
- Piyush Jain ORCID: orcid.org/0000-0002-0471-4663 1 ,
- Scott L. Stephens 6 ,
- Steve W. Taylor 7 &
- Ellen Whitman 1
Communications Earth & Environment volume 4 , Article number: 309 ( 2023 ) Cite this article
15k Accesses
8 Citations
309 Altmetric
Metrics details
- Climate-change impacts
- Ecosystem ecology
- Environmental impact
- Environmental sciences
- Natural hazards
In the province of British Columbia, Canada, four of the most severe wildfire seasons of the last century occurred in the past 7 years: 2017, 2018, 2021, and 2023. To investigate trends in wildfire activity and fire-conducive climate, we conducted an analysis of mapped wildfire perimeters and annual climate data for the period of 1919–2021. Results show that after a century-long decline, fire activity increased from 2005 onwards, coinciding with a sharp reversal in the wetting trend of the 20th century. Even as precipitation levels remain high, moisture deficits have increased due to rapid warming and increased evaporative demand. Bottom-up factors further influence fire activity, as the legacy of past wildfires, insect outbreaks, and land-use practices continually influence fire regimes. The compound effects of climate-induced moisture changes and altered fuels now force British Columbians to confront the harsh reality of more frequent years of intense and prolonged wildfire activity.
Similar content being viewed by others
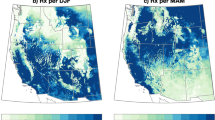
Climate change is narrowing and shifting prescribed fire windows in western United States
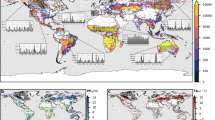
Spatial and temporal expansion of global wildland fire activity in response to climate change
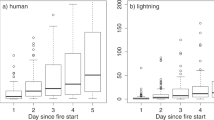
Human-ignited fires result in more extreme fire behavior and ecosystem impacts
Introduction.
The province of British Columbia (BC), Canada, has experienced its four most severe wildfire seasons (2017, 2018, 2021, and 2023) of the last half-century during the past 7 years, all of which were marked by weather extremes. For example, in the summer of 2021 a heat dome that covered most of western Canada shattered temperature records and fueled wildfires from central Canada to the Pacific Coast 1 . The warmest temperature ever recorded north of the 45th parallel (49.6 °C) occurred in the small town of Lytton, BC, on June 29, 2021; the next day, a fast-moving fire that started on the edge of the town spread through and destroyed most of the community in minutes. Dozens of synchronous ignitions from lightning and people in early July led to fire-suppression resources being fully committed. As fire-conducive weather persisted, some uncontained wildfires burned for months, producing dense smoke that covered central and southern BC and spread eastward to central Canada and southward to some northern and midwestern states of the conterminous USA. Some wildfires exhibited extreme behavior, such as fire whirls, as well as the production of lightning from the pyrocumulus clouds that ignited new wildfires 2 . Many homes were lost across BC, and a record number (168) of evacuation orders were issued 3 . First responders grappled with chronic stress and exhaustion. Although the historic events in 2021 came soon after the record-breaking years of 2017 and 2018, many people in and outside of BC were still surprised at their severity. No more than 2 years later, in 2023, wildfires have already burned 1.75 Mha in BC (as of August 24), breaking the previous area-burned record and registering its largest-ever wildfire (Donnie Creek fire, ~550,000 ha).
Compared to other parts of western Canada that are situated mostly in the boreal biome, fire activity in BC in the 20th century was considered low or moderate, with very large wildfires (>50,000 ha) being relatively infrequent. While climate and weather are undoubtedly the major drivers of fire activity in BC, several wide-ranging anthropogenic factors also come into play, notably land-use change and fire-management policies focused on fire suppression 4 , 5 , 6 . These factors, in combination with climate, have shaped wildfire trends over the past century. In the early 20th century, pronounced droughts and numerous human-ignited fires due predominantly to logging, mining, land clearing and railroad construction led to a wildfire regime characterized by numerous fires of relatively small size, on average 7 , 8 . Since the 1950s, the number and area burned of wildfires had been steadily decreasing in the province until ~2000 (277.7 fires ≥20 ha/year and 212,000 ha/year during 1919–1950 vs 116.8 fires and 89,000 ha/year during 1951–2000), a consequence of a cooler and wetter climatic pattern mid-century 9 and increasing fire suppression effort and effectiveness. Contrasting with this 20th-century decline in fire activity, studies investigating future fire potential unanimously project substantial increases in fire activity in BC over the 21st century 10 , 11 . That is, assuming no change in current fire management and land use, the projected warming and drying of the climate would invariably reverse the historical decreasing trend in fire activity and cause a marked amplification of annual area burned rates towards the middle of the century. The recent surge in fire activity is thus, in a sense, unsurprising. What is surprising, however, is the early onset of the increase in wildfire activity around the year 2000—decades earlier than anticipated—and the sheer magnitude of fire-season severity (e.g., three of the past 7 years experienced >1 Mha or >1% of the land area burned; compared to only three wildfire seasons from 1919 to 2016 surpassing 0.5 Mha).
Across its 94.5 Mha (more than twice the area of California), the diverse relief and geology of BC, combined with steep climatic gradients, results in staggering diversity in biological communities and disturbance regimes (Supplementary Fig. 1 ). With a population of approximately five million, densities of people and infrastructure (e.g., permanent roads) are low in much of BC, with most of the large urban centres on the south coast. Its 60 Mha of forests and other natural vegetation types (i.e., grasslands, shrublands, and woodlands) have shaped the area’s social, cultural, and economic identity. BC’s forests essentially span all of the moisture spectrum, from temperate rainforests to high-desert woodlands; approx. 15% are considered dry, 31% wet, and 54% mesic 12 . Broadly, BC can be stratified in three large zones, considered in this study (Fig. 1 ): a Central zone covering a large inland area in the southern two-thirds of the province, a Coastal zone forming a broad band of marine-influenced ecosystems bordering the Pacific Ocean, and a Northern zone that represents a transition to northern forests. All zones are heavily forested but also comprise a non-negligible portion of grasslands (1%) and shrublands (7.2–12.5%); surprisingly, the proportion of agricultural lands is relatively low (<2%) (Table 1 ).
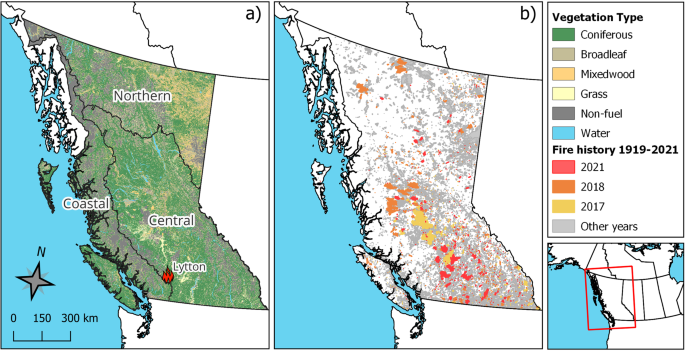
Vegetation type ( a ) from 2019 Risk Analysis Fuels Map (see data and code availability). Wildfire perimeters from the National Burned Area Composite 82 ( b ) emphasizing the 2021, 2018, and 2017 wildfire seasons. The town of Lytton was largely destroyed in a 2021 wildfire.
A land dominated by mountains, the physiography of BC has a strong influence on fire regimes. The Central zone comprises a series of mountain ranges, interspersed with valleys and plateaus. Historically, ecosystems in valleys in the rain shadow of the mountains and plateaus in the southern part of the zone had both surface (i.e., non-lethal to trees) (5–20 years intervals) and stand-replacing wildfires (150–250 years) as part of a mixed-severity wildfire regime. Stand-renewing wildfires were historically frequent (125–175 years) in forests on the central plateaus and infrequent to rare (250–300 years) on the windward western side of the interior ranges and at high elevation 13 , 14 . It has been the most fire-active zone in BC (followed closely by the Northern zone) over the last century and recorded a long history of Indigenous fire use, some areas having low mean fire intervals (e.g., 5–10 years) 15 , 16 . The Coastal zone, falling to the west but including the rugged Coast Range, hosts some highly productive, conifer-dominated forests, including its iconic old-growth forests. Much of the Coastal zone had infrequent stand-replacing fires (150–350 years) historically, with a mix of surface (50–100 years) and stand-replacing (100–300 years) wildfires in forests in the rain shadow of the Vancouver Island Ranges, and rare stand-replacing fires in high-elevation coastal forests (350–450 years) 13 , 14 . While naturally occurring wildfires are uncommon relative to the other two zones, evidence of fire is present throughout the zone and the millennia-long use of cultural burning has shaped ecosystems locally 17 , 18 , 19 . The Northern zone, with contrasting mountainous and relatively flat landscapes in the west and east, respectively, is composed of boreal and sub-boreal ecosystems. Wildfire regimes in this zone are typical for cold forests; that is, characterized by large, high-intensity (i.e., mostly stand-replacing) wildfires. Historically, stand-replacing fires were frequent (50–150) to infrequent (200–350) in the plains and mountains, respectively 13 , 14 . Ample prescribed and Indigenous burning occurs to this day, notably to improve elk, sheep, and bison habitat 20 .
Although natural and anthropogenic factors have modified natural systems in BC for millennia, many fear that the current rate of anthropogenic change will compromise the resilience of natural and human systems 21 . Wildfire represents a strong transformative agent with immense implications for community vulnerability, ecosystem services, and carbon sequestration in BC. In this study, we examine the contemporary trends in wildfire activity in BC and discuss how a century of rapid climatic, demographic, and land-cover change may have contributed to the changing wildfire regimes. We ask: has climate change pushed BC, quickly and suddenly—if not unexpectedly—into a new fire epoch? Specifically, we compare the trends in fire activity over the past century across BC to annual climate variables known to influence fire activity. These trends are considered in the context of future projections of climate. We also discuss how the interplay between climatic and bottom-up (i.e., non-climatic biophysical or anthropogenic) factors, may have shaped contemporary fire regimes in BC. Finally, we discuss the ramifications of the current amplification in fire activity for the people and ecosystems of BC in light of the ongoing anthropogenic climate disruption.
Results and discussion
Top-down controls on bc wildfires: climate.
Strong trends in temperature, precipitation, and an integrated measure of the two, the moisture deficit, have been observed in BC over the past century, and significant changes in the direction or breakpoints of these trends have been identified (Fig. 2 ). Although it is true that climate and fire activity fluctuates across decades to millennia, climates of BC have become more conducive to fire since ~2000 compared to previous decades, despite considerable year-to-year variability. Our results reproduce the previously reported wetting trend until ~2005 9 , but show that the trend in moisture deficit has inverted to a drying one, in both spring (statistical breakpoint in 2011) and summer (breakpoint in 1999) (Fig. 2 ). In addition, the average length of the wildfire season inferred from weather records (the number of frost-free days) and the onset of fire activity (date at which 2% of the year’s total area burned was reached) has increased by 26.7 and 27.1 days, respectively, since the early 20th century. Area burned correlates significantly to the climatic moisture deficit (CMD) (Fig. 3 ) and, accordingly, we observe a concomitant increase in area burned in BC (breakpoint in 2008) after a century-long decrease (Fig. 2 ). In short, even when total precipitation levels remain high, rapid warming results in increased evaporative demand. It was estimated that for every degree of warming, a minimum increase of 15% in precipitation is required to compensate for increased biomass flammability 22 . Our results show a precipitation increase of only 3.34% and 5.74% per degree of warming in spring and summer, respectively, for the period covering the modern rise in temperature (i.e., breakpoints).
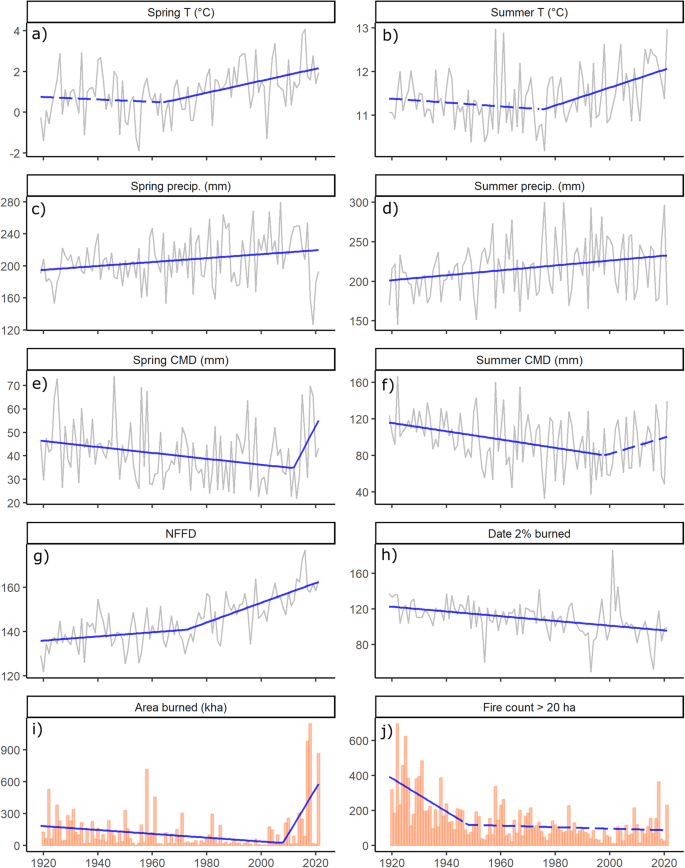
Individual subplots show trends in temperature ( a , b ), total precipitation ( c , d ), climatic moisture deficit (CMD) ( e , f ), number of frost-free days (NFFD) ( g ), date at which 2% of cumulative annual area burned is reached ( h ), annual area burned ( i ), and annual number of fires larger than 20 ha ( j ). Gray lines indicate annual means and blue lines represent segmented regression trendlines. Solid lines indicate a significant trend (one-sided Mann-Kendall test, p < 0.10).
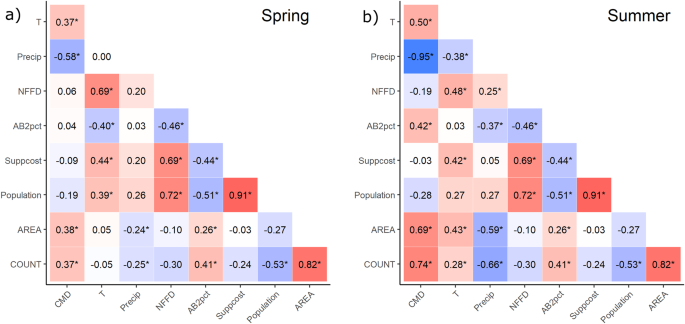
Spring ( a ) and summer ( b ) variables include climatic moisture deficit (CMD), average temperature (T), precipitation (Precip), number of frost-free days (NFFD), the date at which 2% of cumulative annual area burned is reached (AB2pct), annual suppression cost (Suppcost), area burned (AREA), and number of fires over 20 ha (COUNT). Significance ( p < 0.05) of correlations is indicated by *.
A number of studies have pointed to the overriding role of temperature on the recent uptick in fire activity in western North America 23 , 24 and in other parts of the world 25 . However, all aspects of weather, including precipitation, relative humidity, and wind, influence fuel moisture, hence flammability, through their interactions with the relief and vegetation. The outcome of these complex interactions is evidenced in the highly variable fire environments in our study. Over the last century, the Central zone saw an increase in temperature (ΔTemp., 1970–2021 = 0.98 °C), an abrupt decline in summer precipitation, and an increase in summer CMD (Supplementary Fig. 2 ). This zone was also most affected by the recent mountain pine beetle epidemic (below), and experiences frequent synchronous wildfire ignitions, allowing the effects of reduced fuel moisture to translate into increased wildfire activity 26 . Interestingly, the CMD of the Central zone (and of BC) was higher at the beginning of the 20th century, suggesting a drier climate than that of the last decades, though it should be noted that there were few weather stations in inland BC in the early 1900s. Although the Coastal zone experienced the largest increase in temperature (ΔTemp. = 1.25 °C) and increases in spring and summer CMD, it did not see an increase in fire activity (Supplementary Fig. 3 ). Despite the rapidly growing potential for wildfire, contemporary wildfire occurrence is low compared to the first half of the 20th century, a period of heavy industrialization due to extensive logging, land clearing, and mining on the coast (as in the Central zone). The Coastal zone is inherently less flammable than the Central and Northern zones, in part due to ignition limitation (lightning storms are rare) and the tall forests less prone to crown fire. Historically, large fires in coastal forests were often linked to offshore outflow events bringing warm dry air and high winds from the interior, as it occurred in the state of Oregon, USA, the 1933 Great Tillamook Fire 27 and more recently during the 2020 wildfire season 28 . The increasing summer CMD in the Coastal zone suggests a need for heightened alertness for these “fire winds”. The temperature increase in the Northern zone was the lowest of the three zones (ΔTemp. = 0.61 °C), with concomitant increasing trend in summer wetness and lower CMD (Supplementary Fig. 4 ). While a rapid increase in wildfire activity in this zone may not appear imminent, in 2023 the area experienced its most active wildfire season—by far—of the last century, with nearly 1 Mha burned by August 24, compared to the previous annual maximum of approx. 0.4 Mha.
Wildfire ignition and spread is determined primarily by day-to-day weather superimposed on (and also affected by) broad climatic patterns and oscillations including El Niño–Southern Oscillation/Pacific Decadal Oscillation 29 , 30 , making fingerprinting the specific effects of climate change on fire activity challenging. With all else being equal, a climate characterized by more hot, dry, and windy days will invariably lead to more ignitions, faster spreading, longer-burning and, ultimately, larger wildfires 31 . In recent years, the extreme fire-conducive weather in BC led to numerous large wildfires that burned for weeks to months, but are those conditions the outcome of a changing climate? A formal attribution study shows that the fire activity of the 2017 wildfire season can confidently be associated with the recent climate disruption 32 . Unsurprisingly, the massive 2021 heat dome that yielded record temperatures is an almost-certain outcome of human-induced climate change: it is one of the most extreme weather events ever recorded in BC 33 and is estimated to have been 150 times less likely to have occurred without the changing climate 34 . In the western US, Abatzoglou and Williams 35 have convincingly demonstrated that the sharp increase in fire activity is mostly the result of prolonged annual moisture deficits. Coherent with these results, we report significant correlations between fire-activity metrics (area burned and fires ≥20 ha) and several climate variables in BC, from 1919 to 2021 (Fig. 3 ). Given the current and projected climate trajectory, it is likely that the potential for wildfire will continue to increase in the upcoming century, even under the most optimistic climate scenario 11 . According to CMIP6 projections, the brackets of temperature increases are highly coherent among zones, whereas CMD values may vary considerably, from the highest deficit in the Central zone to the lowest in the Northern zone (Fig. 4 ).
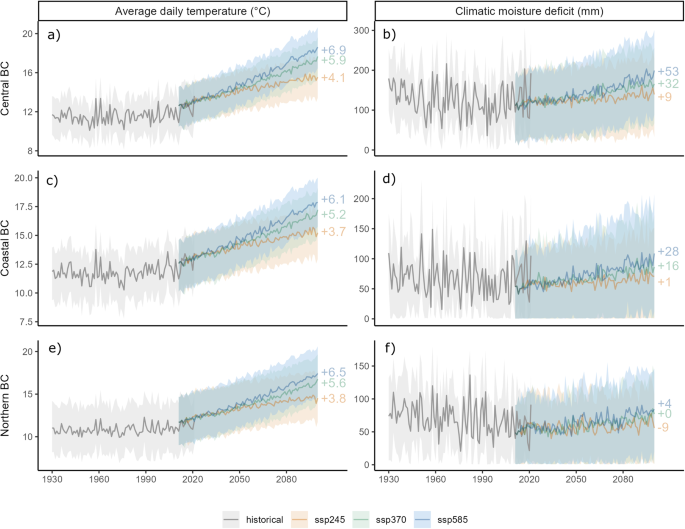
Projections shown are three CMIP6 Shared Socio-economic Pathway (SSP) scenarios (ssp245, ssp370, spp585) 78 , averaged over the Central zone ( a , b ), the Coastal zone ( c , d ), and the Northern zone ( e , f ). Shaded region represents standard deviation of records across the zone of interest.
A recent global analysis depicting the fire weather trends of the last few decades shows that the southern half of BC has experienced a significant increase in extremes of fire-conducive weather, as part of a continuous pattern that is prevalent in much of the western USA 24 . Unlike US states to the south, however, the increasing trend in area burned in BC occurred later (2008 breakpoint) than northwestern states (mid-1980s), the Interior West and Southwest (mid-1980s) 36 , or California (early 20th century) (Supplementary Fig. 5 ). Although the trends in temperature are similar among areas, it is difficult to compare moisture deficits, as the range of values differs greatly among areas; California, for instance, has an average CMD that is about five times greater (i.e., drier) than BC (Supplementary Fig. 5 ). Beyond the changes in the intensity of climate warming and drying, one may wonder if changes in large-scale weather patterns may be influencing fire weather in BC. The possibility of dynamical changes to the jet stream due to rapid arctic warming and an associated increase in midlatitude extreme weather patterns has been suggested 37 , but this connection is not yet well understood 38 . Regardless, even prior to the 2021 heat dome, background warming of the climate was likely increasing both the size and intensity of heat waves that may be linked to wildfire episodes 39 , 40 . When numerous fire ignitions occur during sustained and large-scale weather events, they will yield a large number of out-of-control synchronous wildfires that rapidly overwhelm fire-suppression capabilities 41 . This syndrome perfectly describes the 2017, 2018, 2021—and now, 2023—wildfire seasons in BC. While widespread, synchronous wildfire events have occurred for centuries or more (e.g., 42 ), projections of future ignition rates suggest that they will become more frequent and further undermine fire-protection efforts 43 .
Bottom-up controls: fuels, land-use history, and fire-management policy
Whereas wildfires across the province of BC are largely governed by climate and weather, bottom-up factors further influence fire activity at the local scale. This is particularly evident with respect to fuels, for which the legacy and cumulative impacts of past wildfires, insect outbreaks, and land-use practices (e.g., logging, agriculture, grazing, urban development) have influenced—and continue to do so—the current fire regimes of BC 44 . In addition, substantial evidence of cultural burning in coastal, interior rainforest, and sub-boreal ecosystems across BC bear the imprint of past and current Indigenous fire stewardship. Fires in coastal temperate rainforests have been chiefly human driven, and the effects of fire exclusion were more pronounced in places where human ignitions comprised the majority of fire starts prior to European contact 19 . An illustration of bottom-up impacts is shown in an area of central BC, with a focus around the 2021 Sparks Lake Fire, near the city of Kamloops (Fig. 5 ). Although it is beyond the scope of this study to provide a synthesis of the effects of disturbances on wildfire occurrence, this map illustrates the magnitude and extent of landscape changes that have occurred in the decades prior to the 2021 wildfire season. Along with more accurate climate projections, gaining a better understanding of how natural and anthropogenic disturbances affect subsequent fire ignition and growth on BC landscapes is critical to improving forecasts of future wildfire activity, either for the next season or over the next few decades. This is a formidable task, however, given the diversity of BC’s vegetation types and their complex interactions with climate and disturbance regimes 45 , 46 .
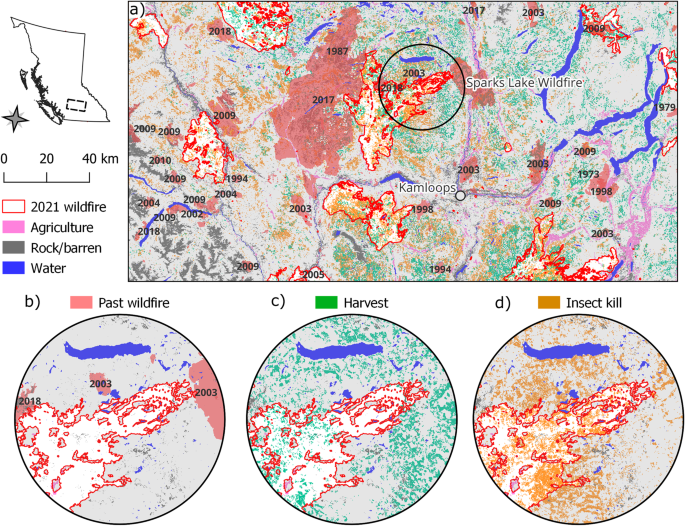
Regional context ( a ) and local context depicting areas that were burned by historic wildfires (1971–2020) ( b ), harvested for forestry purposes (1984–2015) ( c ) 92 , or affected by mountain pine beetle ( Dendroctonus ponderosae ) (1984–2015) ( d ) 92 .
The extent of the most recent mountain pine beetle ( Dendroctonus ponderosae ) outbreak caused extensive mortality over ~15 Mha of forest in BC in the late 1990s and 2000s throughout most of the Central zone and in parts of the Coastal and Northern zones (Table 1 ). The outbreak was aggravated by decades of aggressive fire suppression leading to a shift in age distribution to older, susceptible pine stands, in conjunction with warming winters 47 , 48 . The specific influence of beetle-affected stands on fire occurrence and behavior, while debated elsewhere 49 , are clear in hard-hit areas of BC: fire spread rates are higher in recently attacked stands 50 , whereas severe deadfall later on has challenged fire-management activities 51 , 52 . Although the precise impact of beetle attack is unknown, analysis from the US Pacific Northwest found a 1.61-fold increase in fire likelihood following beetle attack, compared to unaffected stands 53 . More ambiguous are the interactions between forest harvesting and wildfire. BC has a vigorous forest industry (193,000 ha/years harvested, on average, since 1990; https://www.for.gov.bc.ca/ ) with a long (~150 years) history of commercial harvesting in some regions. Forestry practices (e.g., harvesting, salvage logging, replanting, etc.), as well as their effects on wildfire occurrence, vary considerably among regions and forest types. Many areas have been heavily logged and replanted, to a point where management has altered the potential for wildfire occurrence, either negatively, through removing flammable biomass 10 and reducing connectivity between flammable stands or positively by artificially increasing the proportion of forest cover or altering post-harvest species compositions to favor more flammable tree species. For example, forest cover has increased at the expense of grassland and shrublands in some dry forests in southeast BC since the 1950s, a trend that contributes to the current potential for crown fires 54 . Similarly, logging has contributed to structural stand changes that favor wildfire ignition and spread in coastal forest types ill-adapted to intense fire 8 , 17 .
The long-term impact of fire suppression on wildfire potential in North America is increasingly being recognized 6 , 55 . With a fire-management policy that can be described as “hit it hard, hit it fast” over most of its landmass, it seems plausible that decades of successful fire exclusion, combined with the suppression of Indigenous cultural burning practices 56 , have led to a fire deficit in some areas 57 . These policies have contributed to a densification of forest stands relative to the pre-suppression era in parts of BC and, by extension, increased the likelihood of large, high-intensity wildfires 15 , 17 . Deficits are often concentrated around human values we are trying to protect (i.e., communities, parks and protected areas), creating the unintended consequence of increasing fire likelihood 58 and potentially causing profound ecological change. This is the case for many grassland ecosystems and woodlands across BC that were historically maintained through complex Indigenous fire management systems involving frequent cultural fires and lightning-ignited fires. These interactive and generally low-severity fire regimes have been disrupted through decades of fire suppression and human land-use change 17 . Direct fire-suppression costs are continually increasing in tandem with burn rates and a rapidly expanding wildland-urban interface 59 (Supplementary Fig. 6 ). At the same time, the scale of post-harvest fuel mitigation work has decreased significantly over the past three decades. Prior to the early 1990s, prescribed burning (specifically, broadcast burning, as well as burning for wildlife habitat) occurred across tens of thousands of ha/year 3 . By the early 1990s the use of prescribed fire had decreased to less than 10,000 ha/year 3 .
While the direct effects of weather on wildfire ignition and spread are increasingly well understood, the indirect effects of climate change on future wildfire activity shroud our forecasts with massive uncertainty. In south-central BC, it has been suggested that the hot and dry weather that drives large wildfires may also lead to a depletion of flammable biomass 60 . Rapid compositional and structural changes to some forest types due to accelerated tree mortality (i.e., through drought, insect outbreaks, or pathogens) may lead to unanticipated and unpredictable effects on wildfire occurrence and fire behavior 30 , 61 . Even though the paleo-record points to dramatic shifts in fire activity and resulting vegetation in BC over the last few millennia 62 , the current situation is firmly without analog in either the biophysical context or anthropogenic setting, but also due to the rapidity of climatic changes. To counter this uncertainty, a growing interest in climate-smart landscape and land-management strategies for BC show promise in augmenting our ability to adapt to a rapidly changing disturbance regime 63 . One such adaptation strategy consists of manipulating forests to reduce their vulnerability to severe wildfires, bark beetle attacks, and climate change by coupling trees having specific traits with that of novel environments and fire regimes 64 , 65 .
Looking ahead
With four wildfire blowup seasons (2017, 2018, 2021, and 2023) in 7 years—and associated destruction, threats, and hardship to human and ecological communities—BC now appears to have arrived at its place as a hotspot for catastrophic wildfire losses, along with Australia, the western US, and the Mediterranean Basin. As BC shares some of the ecosystems and weather systems that drive many fire regimes of the western US, it is similarly and unequivocally on that same trajectory of fire-regime change, albeit with a delay relative to the inflexion point documented in some coastal states of the western US 36 , 66 (Supplementary Fig. 5 ). Just as British Columbians became accustomed to the relatively low burn rates of the late 20th century, they are now confronted with a harsh reality of more frequent years of intense and prolonged wildfire activity. Moreover, there is no indication that an upward trend in climate-induced wildfire potential will stabilize in the near future, as even the coolest and wettest climate projections point to an increase in moisture deficit until at least the end of the 21st century in many parts of the province 67 , 68 (Fig. 4 ). From a global perspective, the surge in wildfire activity observed in BC is disquieting, especially if it heralds—as projected—similar increases in the neighboring vast, carbon-rich boreal biome 69 . Despite a rapid rise in temperature observed in the adjacent Northwest Territories and Yukon over the last half-century, no significant increases in area burned or moisture deficit have been observed in recent decades (Supplementary Fig. 5 ).
Looking into the past suggests that, while the nature of a modern fire environment may be unique, high annual rates of burning are not unprecedented. Several years prior to the recent string of severe wildfire years in California, Stephens et al. 70 concluded that “[…] prehistorically a large amount of California burned every year”. In fact, their multi-proxy reconstruction suggests that the burn rates from 1950–1999 constituted a mere 5.6% of the European pre-settlement rates, a decrease mainly resulting from the exclusion of Indigenous cultural burning practices and widespread land-cover change (mainly agricultural and urban). Since the publication of that article, the state, as well as much of the western US, has endured the dramatic climate-induced increase in fire activity that is further exacerbated by a lengthening of the wildfire season caused by the numerous human-caused fires throughout the warm months 71 . BC may be in a similar historic fire-deficit situation, as reported by Smith 72 , who suggests a possible ten-fold decrease in contemporary burn rates compared to those of the European pre-settlement period. While it is difficult to interpret the relevance of these historical burn rates in today’s reality, it underscores the often-underestimated burning potential of fire-prone landscapes and opens the door to further discussions on how to better coexist with fire 73 .
The recent amplification in the fire regimes of BC, in conjunction with its ever-expanding wildland-urban interface, bear many consequences for British Columbians. The steady rise in community evacuation orders and evacuees come with a heavy human and economic cost (Supplementary Fig. 7 ). The population of BC has mourned the loss of civilians and fire fighters, experienced significant destruction of homes and forest values, endured enormous disruption caused by evacuations, and suffered exposure to harmful chemicals from smoke. Yet, we are only beginning to understand the effects of wildfires on people’s mental and physical health 74 , 75 , 76 . Cascading secondary effects of wildfires, such as debris flow, flooding, and mudslides can also be devastating, as shown in the years following the 2003 wildfires in south-central BC 77 . Destructive floods covered much of central BC a few months after the 2021 wildfires, but it is still unclear how much of a role wildfires may have played in such a large-scale event 78 . In July 2022, a wildfire two kilometers west of Lytton destroyed several houses almost a year after burning down the town, serving as a clear reminder that as long as there is flammable vegetation and hot, dry, windy weather, a wildfire may ignite and spread.
Though daunting, the current conjuncture in BC provides a strong impetus for accelerating efforts towards fire adaptation and mitigation that protect human communities and maintain essential ecosystem services within a broader climate change adaptation context 79 . To take on this task, many tools are available: landscape fire management plans enabling prescribed burning, Indigenous-led cultural burning, fuel mitigation treatments, optimization of forest harvesting, and species conversions, as well as revised urban planning and building codes and practices, and enhancing preparedness and resilience in fire and emergency organizations. Mitigation strategies such as fuel treatments must, however, be tailored to the diverse vegetation types and wildfire regimes of BC; for instance, prescribed burning and tree thinning may be appropriate for dry forests, but other strategies should be considered for moist coastal areas (e.g., retention and promotion of old-growth features) or boreal forests (e.g., harvesting, broadleaf-species conversion) 80 , 81 . It is becoming evident that we require place-based efforts across firescapes that are both creative and sustained to confront the magnitude of the wildfire challenge in different socioeconomic and ecological situations across BC. This may involve a philosophical change in the way we think about wildfires to an outlook accepting more wildland fire where it makes sense (i.e., for protection or ecological purposes) while emphasizing people’s role in proactively managing fire-prone landscapes 82 . Our ability to respond to rapid and unsettling changes in our fire environment must overcome our cognitive dissonance or normalcy biases. To counter this, a critical step in adapting to fire is to recognize and accept that BC has entered a new and uncertain fire epoch.
Climate and fire data
We summarize historical and projected climate data from 1900 to 2100 for BC and three nested zones (Fig. 1 ), as well as five selected US states and Canadian provinces. These climate data are interpolated using ClimateNA v7.31 83 at a 50-km resolution, including three WorldClim CMIP6 Shared Socio-economic Pathway (SSP) scenarios (ssp245, ssp370, spp585) from 2021 to 2100 84 . To limit collinearity, we selected a set of variables having | r | < 0.7 (Spearman correlation): seasonal mean temperature, total precipitation, and Hargreaves CMD for spring (March, April, May) and summer (June, July, August), and the annual number of frost-free days. We measured the association among variables using a Spearman correlation test modified for serially correlated data 85 (Fig. 2 ) implemented in the astrochron R package 86 .
Mapped wildfire perimeters were drawn from the Canadian National Fire Database for 1919–1984 87 and the National Burned Area Composite 88 for 1985–2021. We adjust fire polygon burned area estimates to compensate for missing unburned islands and perimeter inaccuracies arising from less-accurate sources such as manual delineation or GPS perimeters 89 . We excluded small fires (<20 ha) from this dataset, which were not consistently reported over the study period. We calculated total area burned per year, number of fires per year, and day-of-year at which cumulative area burned reaches 1%, 2%, 5%, and 10% of the annual total (the 2% threshold was retained).
Fire statistics for areas outside BC were summarized using the Canadian National Fire Database 87 fire occurrence data for Yukon and the Northwest Territories, the Fire and Resource Assessment Program historical polygons for California, and the Monitoring Trends in Burn Severity fire polygons for Washington and Oregon 90 .
Fire suppression costs and evacuations
Direct fire suppression costs were compiled from BC Ministry of Forests, Lands, Natural Resource Operations and Rural Development Annual Reports 91 for 1919–1969, Stocks and Martell 92 for 1970–2009, and BC Wildfire Service Annual Reports 93 for 2010–2021. All costs were adjusted for inflation based on the historical consumer price index 94 . Data on evacuations were drawn from the Canadian Wildland Fire Evacuation Database (see data availability).
Trend analysis
All climate and fire variables were plotted as a time series for 1919–2021. We used the “segmented” function from the segmented R package 95 to estimate segmented linear relationships for all variables across the study period (Fig. 3 ). This uses maximum likelihood estimation to test whether piecewise linear regression of two (or more) parts better fits the data than normal linear regression, and estimates breakpoints and slopes for each component part of the segmented regression. We tested the statistical significance of segmented trends using a modified Mann-Kendall trend test, with a variance correction for serially correlated data 96 , available from the modifiedmk R package 97 .
Forest disturbance maps and statistics
Harvested cutblocks and areas affected by the mountain pine beetle ( Dendroctonus ponderosae ) epidemic of the 1990s and early 2000s were obtained from the CanLAD dataset 98 , which is a 30-m resolution satellite-derived dataset of land cover change for the period of 1986–2020. The fire history of the area, from 1919 to 2021 was mapped from the previously mentioned fire polygon datasets, and cropland was mapped using the 30-m resolution 2010 Canada Land Cover dataset 99 .
We summarize spatial statistics of forest disturbance and land cover by provincial and ecozone averages (Table 1 ). Land cover statistics are summarized from SCANFi land cover dataset, a version of the CanLAD dataset family 100 .
Data availability
The datasets generated during this study are available at the Centre for Open Science OSF data repository [ https://doi.org/10.17605/OSF.IO/2G3QK ] 101 . All climate data was accessed and interpolated using ClimateNA v7.31, available at https://climatena.ca/ . Fire perimeters and point data for Canada were downloaded from the Canadian Wildland Fire Information System Datamart at https://cwfis.cfs.nrcan.gc.ca/datamart . Fire polygon data for California were downloaded from the Fire and Resource Assessment Project at https://www.fire.ca.gov/what-we-do/fire-resource-assessment-program . Fire polygon data for Oregon and Washington were downloaded from the Monitoring Trends in Burn Severity Project at https://www.mtbs.gov/ . Although the Canadian Wildland Fire Evacuation Database is not publically-accessible, BC-specific data summarized from this database are included in the OSF data repository. tPublic road shapefiles, used for regional statistics, are available from the CanVec dataset https://open.canada.ca/data/en/dataset/2dac78ba-8543-48a6-8f07-faeef56f9895 . BC cutblock shapefiles, used for regional statistics, are available from https://catalog.data.gov.bc.ca/dataset/harvested-areas-of-bc-consolidated-cutblocks - . Vegetation cover maps for Study Area figure accessed at https://cwfis.cfs.nrcan.gc.ca/downloads/fuels/development/ . Mountain Pine Beetle impact extent was calculated from polygons available at the BC Government Ministry of Forests data portal, https://www.for.gov.bc.ca/ftp/HFP/external/!publish/Aerial_Overview/ .
Code availability
All analysis was conducted in R version 4.2.2. Specialized R packages used in the analysis include astrochron 86 , segmented 95 , and modifiedmk 97 . Code and data for replicating the analysis are archived at the Centre for Open Science OSF data repository [ https://doi.org/10.17605/OSF.IO/2G3QK ] 101 .
White, R. H. et al. The unprecedented Pacific Northwest heatwave of June 2021. Nat. Comm. 14 , 727 (2023).
CAS Google Scholar
Peterson, D. A. et al. Wildfire-driven thunderstorms cause a volcano-like stratospheric injection of smoke. NPJ Clim. Atmos. Sci. 1 , 30 (2018).
Google Scholar
Hoffman, K. M., Christianson, A. C., Gray, R. W. & Daniels, L. Western Canada’s new wildfire reality needs a new approach to fire management. Environ. Res. Lett. 17 , 061001 (2022).
Meyn, A. et al. Spatial variation of trends in wildfire and summer drought in British Columbia, Canada, 1920–2000. Int. J. Wildland Fire 19 , 272–283 (2010).
Marcoux, H. M. et al. Differentiating mixed-and high-severity fire regimes in mixed-conifer forests of the Canadian Cordillera. For. Ecol. Manag. 341 , 45–58 (2015).
Copes-Gerbitz, K., Hagerman, S. M. & Daniels, L. D. Transforming fire governance in British Columbia, Canada: an emerging vision for coexisting with fire. Reg. Environ. Change 22 , 48 (2022).
Johnson, E. A., Fryer, G. I. & Heathcott, M. J. The influence of man and climate on frequency of fire in the interior wet belt forest, British Columbia. J. Ecol . 82 , 403–412 (1990).
Pew, K. L. & Larsen, C. P. S. GIS analysis of spatial and temporal patterns of human-caused wildfires in the temperate rain forest of vancouver island, Canada. For. Ecol. Manag. 140 , 1–18 (2001).
Meyn, A. et al. Precipitation-driven decrease in wildfires in British Columbia. Reg. Environ. Change 13 , 165–177 (2013).
Nitschke, C. R. & Innes, J. L. Climatic change and fire potential in south‐central British Columbia, Canada. Glob. Chang. Biol 14 , 841–855 (2008).
Wang, X. et al. Projected changes in daily fire spread across Canada over the next century. Environ. Res. Lett. 12 , 025005 (2017).
British Columbia, Ministry of Forests. 1994. Forest, Range, & Recreation Resource Analysis. (Ministry of Forests, 1995).
Parminter, J. V. An historical review of forest fire management in British Columbia. An Essay Submitted in Partial Fulfillment of the requirements for the degree of Master of Forestry in the Department of Forestry, Univ. B.C., Vancouver, B.C. (1978).
Wong, C., Dorner, B. & Sandmann, H. Estimating historical variability of natural disturbances in British Columbia. BC Ministry of Forests & Ministry of Sustainable Resource Management, Resource Planning Branch, Victoria, B.C. Land Management Handbook No. 53 . (2003).
Harvey, J. E., Smith, D. J. & Veblen, T. T. Mixed‐severity fire history at a forest–grassland ecotone in west central British Columbia, Canada. Ecol. Appl. 27 , 1746–1760 (2017).
Brookes, W., Daniels, L. D., Copes-Gerbitz, K., Baron, J. N. & Carroll, A. L. A disrupted historical fire regime in central British Columbia. Front. Ecol. Evol. 9 , 676961 (2021).
Daniels, L. D. & Gray, R. W. Disturbance regimes in coastal British Columbia. J. Ecosyst. Manag . 7 , 44–56 (2006).
Gedalof, Z. E., Pellatt, M. & Smith, D. J. From prairie to forest: three centuries of environmental change at Rocky Point, Vancouver Island, British Columbia. Northwest Sci 80 , 34–46 (2006).
Hoffman, K. M., Lertzman, K. P. & Starzomski, B. M. Ecological legacies of anthropogenic burning in a British Columbia coastal temperate rain forest. J. Biogeogr. 44 , 2903–2915 (2017).
Christianson, A. C. et al. Centering Indigenous voices: The role of fire in the Boreal Forest of North America. Curr. For. Rep. 8 , 257–276 (2022).
Copes-Gerbitz, K. et al. Community engagement with proactive wildfire management in British Columbia, Canada: perceptions, preferences, and barriers to action. Front. For. Glob. Change 5 , 829125 (2022).
Flannigan, M. D. et al. Fuel moisture sensitivity to temperature and precipitation: climate change implications. Clim. Change 134 , 59–71 (2016).
Jolly, W. M. et al. Climate-induced variations in global wildfire danger from 1979 to 2013. Nat. Comm. 6 , 7537 (2015).
Jain, P., Castellanos-Acuna, D., Coogan, S. C., Abatzoglou, J. T. & Flannigan, M. D. Observed increases in extreme fire weather driven by atmospheric humidity and temperature. Nat. Clim. Change 12 , 63–70 (2022).
Abatzoglou, J. T., Williams, A. P. & Barbero, R. Global emergence of anthropogenic climate change in fire weather indices. Geophys. Res. Lett. 46 , 326–336 (2019).
Baron, J. N., Gergel, S. E., Hessburg, P. F. & Daniels, L. D. A century of transformation: fire regime transitions from 1919 to 2019 in southeastern British Columbia, Canada. Landsc. Ecol. 37 , 2707–2727 (2022).
Dague, C. I. The weather of the great tillamook, Oreg., fire of August 1933. Mon. Weather Rev. 62 , 227–231 (1934).
Abatzoglou, J. T., Rupp, D. E., O’Neill, L. W. & Sadegh, M. Compound extremes drive the western Oregon wildfires of September 2020. Geophys. Res. Lett. 48 , e2021GL092520 (2021).
Meyn, A., Taylor, S. W., Flannigan, M. D., Thonicke, K. & Cramer, W. Relationship between fire, climate oscillations, and drought in British Columbia, Canada, 1920–2000. Glob. Change Biol. 16 , 977–989 (2010).
Daniels, L. D. et al. Direct and indirect impacts of climate change on forests: three case studies from British Columbia. Can. J. Plant Pathol. 33 , 108–116 (2011).
Xi, D. D., Taylor, S. W., Woolford, D. G. & Dean, C. B. Statistical models of key components of wildfire risk. Annu. Rev. Stat. Appl. 6 , 197–222 (2019).
Kirchmeier‐Young, M. C., Gillett, N. P., Zwiers, F. W., Cannon, A. J. & Anslow, F. S. Attribution of the influence of human‐induced climate change on an extreme fire season. Earth’s Future 7 , 2–10 (2019).
Thompson, V. et al. The 2021 western North America heat wave among the most extreme events ever recorded globally. Sci. Adv. 8 , eabm6860 (2022).
Philip, S. Y. et al. Rapid attribution analysis of the extraordinary heatwave on the Pacific Coast of the US and Canada June 2021. Earth. Syst. Dyn . 13 , 1–34 (2021).
Abatzoglou, J. T. & Williams, A. P. Impact of anthropogenic climate change on wildfire across western US forests. Proc. Natl. Acad. Sci. USA 113 , 11770–11775 (2016).
Higuera, P. E., Abatzoglou, J. T., Littell, J. S. & Morgan, P. The changing strength and nature of fire-climate relationships in the northern Rocky Mountains, USA, 1902-2008. PloS ONE 10 , e0127563 (2015).
Francis, J. A. & Vavrus, S. J. Evidence for a wavier jet stream in response to rapid Arctic warming. Environ. Res. Lett. 10 , 014005 (2015).
Blackport, R. & Screen, J. A. Weakened evidence for mid-latitude impacts of Arctic warming. Nat. Clim. Change. 10 , 1065–1066 (2020).
Rogers, C. D., Kornhuber, K., Perkins-Kirkpatrick, S. E., Loikith, P. C. & Singh, D. Sixfold increase in historical Northern Hemisphere concurrent large heatwaves driven by warming and changing atmospheric circulations. J. Clim 35 , 1063–1078 (2022).
Sharma, A. R., Jain, P., Abatzoglou, J. T. & Flannigan, M. Persistent positive anomalies in geopotential heights promote wildfires in western North America. J. Clim. 35 , 2867–2884 (2022).
Abatzoglou, J. T., Juang, C. S., Williams, A. P., Kolden, C. A. & Westerling, A. L. Increasing synchronous fire danger in forests of the western United States. Geophys. Res. Lett. 48 , e2020GL091377 (2021).
Chavardès, R. D. et al. Regional drought synchronised historical fires in dry forests of the Montane Cordillera Ecozone, Canada. Int. J. Wildland Fire 31 , 67–80 (2021).
Wotton, B. M., Nock, C. A. & Flannigan, M. D. Forest fire occurrence and climate change in Canada. Int. J. Wildland Fire 19 , 253–271 (2010).
Hamilton, N. P. & Burton, P. J. Wildfire disturbance reveals evidence of ecosystem resilience and precariousness in a forest–grassland mosaic. Ecosphere 14 , e4460 (2023).
Camp, P. E. & Krawchuk, M. A. Spatially varying constraints of human-caused fire occurrence in British Columbia, Canada. Int. J. Wildland Fire 26 , 219–229 (2017).
Nadeem, K., Taylor, S. W., Woolford, D. G. & Dean, C. B. Mesoscale spatiotemporal predictive models of daily human-and lightning-caused wildland fire occurrence in British Columbia. Int. J. Wildland Fire 29 , 11–27 (2019).
Taylor, S. W. & Carroll, A. L. Disturbance, forest age, and mountain pine beetle outbreak dynamics in BC: a historical perspective. In Proc. Mountain Pine Beetle Symposium: Challenges and Solutions. Vol. 3031. (Natural Resources Canada, Canadian Forest Service, Pacific Forestry Centre Victoria, 2003).
Axelson, J. N., Alfaro, R. I. & Hawkes, B. C. Changes in stand structure in uneven-aged lodgepole pine stands impacted by mountain pine beetle epidemics and fires in central British Columbia. For. Chron. 86 , 87–99 (2010).
Romualdi, D. C., Wilkinson, S. L. & James, P. M. A. On the limited consensus of mountain pine beetle impacts on wildfire. Landsc. Ecol . 38 , 1–20 (2023).
Perrakis, D. D., Lanoville, R. A., Taylor, S. W. & Hicks, D. Modeling wildfire spread in mountain pine beetle-affected forest stands, British Columbia, Canada. Fire Ecol. 10 , 10–35 (2014).
Page, W. G., Alexander, M. E. & Jenkins, M. J. Wildfire’s resistance to control in mountain pine beetle-attacked lodgepole pine forests. For. Chron. 89 , 783–794 (2013).
Moriarty, K., Cheng, A. S., Hoffman, C. M., Cottrell, S. P. & Alexander, M. E. Firefighter observations of “surprising” fire behavior in Mountain Pine Beetle-attacked lodgepole pine forests. Fire 2 , 34 (2019).
Meigs, G. W., Kennedy, R. E., Gray, A. N. & Gregory, M. J. Spatiotemporal dynamics of recent mountain pine beetle and western spruce budworm outbreaks across the Pacific Northwest Region. USA. For. Ecol. Manag. 339 , 71–86 (2015).
Taylor, S. W., Baxter, G. J. & Hawkes, B. C. Modeling the effects of forest succession on fire behavior potential in southeastern British Columbia. In Proc. III International Conference on Forest Fire Research, 14th Conference on Fire and Forest Meteorology . Vol. II., 2059–2072 (Luso, Portugal, 1998).
Calkin, D. E., Cohen, J. D., Finney, M. A. & Thompson, M. P. How risk management can prevent future wildfire disasters in the wildland-urban interface. Proc. Natl. Acad. Sci. USA 111 , 746–751 (2014).
Lewis, M., Christianson, A. & Spinks, M. Return to flame: reasons for burning in Lytton First Nation, British Columbia. J. For. 116 , 143–150 (2018).
Parks, S. A. et al. Wildland fire deficit and surplus in the western United States, 1984–2012. Ecosphere 6 , 1–13 (2015).
Parisien, M. A. et al. Fire deficit increases wildfire risk for many communities in the Canadian boreal forest. Nat. Comm. 11 , 1–9 (2020).
Johnston, L. M. & Flannigan, M. D. Mapping Canadian wildland fire interface areas. Int. J. Wildland Fire 27 , 1–14 (2017).
Wang, X. et al. Future burn probability in south-central British Columbia. Int. J. Wildland Fire 25 , 200–212 (2016).
Loehman, R. A., Keane, R. E., Holsinger, L. M. & Wu, Z. Interactions of landscape disturbances and climate change dictate ecological pattern and process: spatial modeling of wildfire, insect, and disease dynamics under future climates. Landsc. Ecol. 32 , 1447–1459 (2017).
Gavin, D. G., Hu, F. S., Lertzman, K. & Corbett, P. Weak climatic control of stand‐scale fire history during the late Holocene. Ecol. 87 , 1722–1732 (2006).
McLaughlin, B. C. et al. Conservation strategies for the climate crisis: an update on three decades of biodiversity management recommendations from science. Biol. Conserv. 268 , 109497 (2002).
Stephens, S. L. et al. Temperate and boreal forest mega‐fires: characteristics and challenges. Front. Ecol. Environ. 12 , 115–122 (2014).
Stevens, J. T., Kling, M. M., Schwilk, D. W., Varner, J. M. & Kane, J. M. Biogeography of fire regimes in western US conifer forests: a trait‐based approach. Glob. Ecol. Biogeogr. 29 , 944–955 (2020).
Westerling, A. L., Hidalgo, H. G., Cayan, D. R. & Swetnam, T. W. Warming and earlier spring increase western US forest wildfire activity. Science 313 , 940–943 (2006).
Haughian, S. R., Burton, P. J., Taylor, S. W. & Curry, C. Expected effects of climate change on forest disturbance regimes in British Columbia. J. Ecosyst. Manag ., 13 , 1–24 (2012).
Kitzberger, T., Falk, D. A., Westerling, A. L. & Swetnam, T. W. Direct and indirect climate controls predict heterogeneous early-mid 21st century wildfire burned area across western and boreal North America. PloS ONE 12 , e0188486 (2017).
Flannigan, M. D., Krawchuk, M. A., de Groot, W. J., Wotton, B. M. & Gowman, L. M. Implications of changing climate for global wildland fire. Int. J. Wildland Fire 18 , 483–507 (2009).
Stephens, S. L., Martin, R. E. & Clinton, N. E. Prehistoric fire area and emissions from California’s forests, woodlands, shrublands, and grasslands. For. Ecol. Manag. 251 , 205–216 (2007).
Balch, J. K. et al. Human-started wildfires expand the fire niche across the United States. Proc. Natl. Acad. Sci. USA 114 , 2946–2951 (2017).
Smith, J. H. G. Fire cycles and management alternatives. In Proceedings of Fire regimes and ecosystem properties, December 11-15, 1978. Honolulu, Hawaii. USDA Forest Service General Technical Report WO-26 , Washington (1981).
Lake, F. K. & Christianson, A. C. Indigenous fire stewardship. In Encyclopedia of Wildfires and Wildland-urban Interface (WUI) Fires . 714-722 (Springer International Publishing, 2020).
Dodd, W. et al. Lived experience of a record wildfire season in the Northwest Territories, Canada. Can. J. Public Health 109 , 327–337 (2018).
Matz, C. J. et al. Health impact analysis of PM2.5 from wildfire smoke in Canada (2013–2015, 2017–2018). Sci. Total Environ. 725 , 138506 (2020).
Grant, E. & Runkle, J. D. Long-term health effects of wildfire exposure: a scoping review. J. Clim. Change Health 6 , 100110 (2022).
Jordan, P. Post-wildfire debris flows in southern British Columbia, Canada. Int. J. Wildland Fire 25 , 322–336 (2015).
Gillett, N. P. et al. Human influence on the 2021 British Columbia floods. Weather Clim . Extremes 36 , 100441 (2022).
Prichard, S. J. et al. Adapting western North American forests to climate change and wildfires: 10 common questions. Ecol. Appl. 31 , e02433 (2021).
Stephens, S. L. et al. The effects of forest fuel-reduction treatments in the United States. BioScience 62 , 549–560 (2012).
McKinney, S. T., Abrahamson, I., Jain, T. & Anderson, N. A systematic review of empirical evidence for landscape-level fuel treatment effectiveness. Fire Ecol. 18 , 21 (2022).
Taylor, S. W., Daniels, L., Copes-Gerbitz, K. & Forbes, K. Wildfires . In S. Safaie and Dercole, F. (Eds.), Resilient Pathways Report: Co-creating new Knowledge for Understanding Risk and Resilience in BC . Government of Canada Natural Resources Canada (2022).
Wang, T., Hamann, A., Spittlehouse, D. & Carroll, C. Locally downscaled and spatially customizable climate data for historical and future periods for North America. PloS ONE 11 , e0156720 (2016).
O’Neill, B. C. et al. The scenario model intercomparison project (ScenarioMIP) for CMIP6. Geosci. Model Dev. 9 , 3461–3482 (2016).
Ebisuzaki, W. A method to estimate the statistical significance of a correlation when the data are serially correlated. J. Clim. 10 , 2147–2153 (1997).
Meyers, S. R. Astrochron: An R Package for Astrochronology. https://cran.r-project.org/package=astrochron (2023).
Parisien, M. A. et al. Spatial patterns of forest fires in Canada 1980–1999. Int. J. Wildland Fire 15 , 361–374 (2006).
Hall, R. J. et al. Generating annual estimates of forest fire disturbance in Canada: the National Burned Area Composite. Int. J. Wildland Fire 29 , 878–891 (2020).
Skakun, R., Whitman, E., Little, J. M. & Parisien, M. A. Area burned adjustments to historical wildland fires in Canada. Environ. Res. Lett. 16 , 064014 (2021).
Eidenshink, J. et al. A project for monitoring trends in burn severity. Fire Ecol. 3 , 3–21 (2007).
British Columbia, Ministry of Forests, Lands, Natural Resource Operations and Rural Development. 2021. Annual Report, 1919–1969. Victoria, B.C., B.C. Ministry of Forests, Lands, Natural Resource Operations and Rural Development. Print. Compiled by John Parminter.
Stocks, B. J. & Martell, D. L. Forest fire management expenditures in Canada: 1970–2013. For. Chron. 92 , 298–306 (2016).
British Columbia, B.C. Wildfire Service. Wildfire Season Summary, 2010–2021. [B.C. Ministry of Forests, Lands, Natural Resource Operations and Rural Development]. https://www2.gov.bc.ca/gov/content/safety/wildfire-status/about-bcws/wildfire-history/wildfire-season-summary (2021).
Statistics Canada. Table 18-10-0005-01 Consumer Price Index, annual average, not seasonally adjusted . (2021).
Muggeo, V. Segmented: an R package to fit regression models with broken-line relationships. R News 8 , 20–25 (2008).
Hamed, K. H. & Rao, A. R. A modified Mann-Kendall trend test for autocorrelated data. J. Hydrol. 204 , 182–196 (1998).
Patakamuri, S. K. & O’Brien, N. modifiedmk: Modified Versions of Mann Kendall and Spearman’s Rho Trend Tests. R package version 1.6. https://cran.r-project.org/web/packages/modifiedmk/ . (2021).
Guindon, L. et al. Missing forest cover gains in boreal forests explained. Ecosphere 9 , e02094 (2018).
Latifovic, R., Pouliot, D. & Olthof, I. Circa 2010 land cover of Canada: local optimization methodology and product development. Remote Sens 9 , 1098 (2017).
Guindon, L. et al. Trends in wildfire burn severity across Canada, 1985 to 2015. Can. J. For. Res. 51 , 1230–1244 (2021).
Barber, Q. E., Parisien, M. & Jain, P. The abrupt, climate-induced increase in wildfires in British Columbia since the mid-2000s; supporting data and code. https://doi.org/10.17605/OSF.IO/2G3QK . (2023).
Download references
Acknowledgements
We thank Andreas Hamann for his assistance with preliminary model inputs and interpretation of mid-century climate trends. M.-A.P., Q.E.B., P.J., S.G.T. and E.W. acknowledge funding from Natural Resources Canada as part of the work towards the Emergency Management Strategy for Canada.
Author information
Authors and affiliations.
Northern Forestry Centre, Canadian Forest Service, Natural Resources Canada, Edmonton, AB, Canada
Marc-André Parisien, Quinn E. Barber, Piyush Jain & Ellen Whitman
Department of Earth, Environmental and Geographic Sciences, University of British Columbia Okanagan, Kelowna, BC, Canada
Mathieu L. Bourbonnais
Faculty of Forestry, University of British Columbia, Vancouver, BC, Canada
Lori D. Daniels & Kira M. Hoffman
Natural Resource Science, Thompson Rivers University, Kamloops, BC, Canada
Mike D. Flannigan
R.W. Gray Consulting Ltd, Chilliwack, BC, Canada
Robert W. Gray
Department of Environmental Science, Policy, and Management, University of California, Berkeley, Berkeley, CA, USA
Scott L. Stephens
Pacific Forestry Centre, Canadian Forest Service, Natural Resources Canada, Victoria, BC, Canada
Steve W. Taylor
You can also search for this author in PubMed Google Scholar
Contributions
M.-A.P., Q.E.B., P.J. and E.W. designed the paper with help from M.L.B, L.D., M.D.F., R.G., K.M.H., S.L.S. and S.W.T.; Q.E.B. analyzed data with input from M.-A.P., Q.E.B., P.J. and E.W. and M.-A.P. led the writing in collaboration with Q.E.B., P.J. and E.W. All authors read and edited this manuscript.
Corresponding author
Correspondence to Marc-André Parisien .
Ethics declarations
Competing interests.
The authors declare no competing interests.
Peer review
Peer review information.
Communications Earth & Environment thanks the anonymous reviewers for their contribution to the peer review of this work. Primary Handling Editor: Yongqiang Liu and Aliénor Lavergne.
Additional information
Publisher’s note Springer Nature remains neutral with regard to jurisdictional claims in published maps and institutional affiliations.
Supplementary information
Supplementary information, rights and permissions.
Open Access This article is licensed under a Creative Commons Attribution 4.0 International License, which permits use, sharing, adaptation, distribution and reproduction in any medium or format, as long as you give appropriate credit to the original author(s) and the source, provide a link to the Creative Commons license, and indicate if changes were made. The images or other third party material in this article are included in the article’s Creative Commons license, unless indicated otherwise in a credit line to the material. If material is not included in the article’s Creative Commons license and your intended use is not permitted by statutory regulation or exceeds the permitted use, you will need to obtain permission directly from the copyright holder. To view a copy of this license, visit http://creativecommons.org/licenses/by/4.0/ .
Reprints and permissions
About this article
Cite this article.
Parisien, MA., Barber, Q.E., Bourbonnais, M.L. et al. Abrupt, climate-induced increase in wildfires in British Columbia since the mid-2000s. Commun Earth Environ 4 , 309 (2023). https://doi.org/10.1038/s43247-023-00977-1
Download citation
Received : 15 November 2022
Accepted : 25 August 2023
Published : 05 September 2023
DOI : https://doi.org/10.1038/s43247-023-00977-1
Share this article
Anyone you share the following link with will be able to read this content:
Sorry, a shareable link is not currently available for this article.
Provided by the Springer Nature SharedIt content-sharing initiative
This article is cited by
How do canadian faculty members imagine future teaching and learning modalities.
- George Veletsianos
- Nicole Johnson
- Shandell Houlden
Educational technology research and development (2024)
By submitting a comment you agree to abide by our Terms and Community Guidelines . If you find something abusive or that does not comply with our terms or guidelines please flag it as inappropriate.
Quick links
- Explore articles by subject
- Guide to authors
- Editorial policies
Sign up for the Nature Briefing: Anthropocene newsletter — what matters in anthropocene research, free to your inbox weekly.


Amazon Rainforest Fires
A staggering 26.4 million acres of brazil’s amazon were scorched in 2023, a 35.4% increase from the previous year, deforestation from agriculture and cattle-ranching is a main driver of forest fires in the amazon, expanding and securing land rights of indigenous peoples is one of the best ways to protect the amazon, rainforest foundation us’s response to the amazon fires.
Healthy communities can manage and protect their lands better than anyone. Building on 35 years of steady, dedicated work with Indigenous partners in the Amazon, Rainforest Foundation US provides direct technical support in legal defense, land titling, monitoring, and institutional strengthening, so that Indigenous communities may continue to manage their rainforest territories with the knowledge and care they have sustained for thousands of years.
Throughout the Amazon, we are supporting partners to actively prevent and respond to the threat of fires by:
- Advancing land rights to over 7.9 million acres of Indigenous peoples’ territories in the Amazon regions of Peru, Brazil, and Guyana.
- Expanding Indigenous monitoring programs—some similar to our Rainforest Alert program in Peru—to over 17.5 million acres of tropical forest throughout Central and South America.
- Launching five high-profile advocacy and legal initiatives to improve territorial security for over 34.6 million acres of land and forests.
How do Indigenous Peoples and Land Rights help prevent fires?
Indigenous peoples are the most effective forest stewards . Rainforests held by Indigenous peoples have a lower presence of fire and lower fire temperatures, meaning they’re better able to resist forest loss. Data also shows that rainforests managed by Indigenous peoples contain greater carbon density than state-managed forests and foster higher levels of biodiversity.
It’s simple: One of the best ways to protect the Amazon from destruction from fire, mining, industrial ranching, and illegal-logging is to secure and expand the land rights of Indigenous peoples living in these territories. Ensuring Indigenous peoples and local communities have rightful governance and control over their territories, as well as access to the necessary technology, training, and resources to manage and protect their territories is crucial to preventing fires and protecting the biodiversity and cultural heritage of the region.
The satellite map below displays real-time fires and Indigenous peoples’ lands. The majority of fires are observed outside Indigenous territories, typically ceasing at their boundaries.,
An area the size of the state of Tennessee burned in the Brazilian Amazon in 2023
While deforestation in the Amazon decreased significantly last year, the forest continued to burn at an alarming rate. For the first time since 2018, the clearance rate was less than 10,000 sq km in the 12 months until 31 July. Still more encouraging, the loss of tree cover in Indigenous territories fell by 73%. Despite these statistics, a staggering 26.4 million acres (10.7 million hectares) of Brazil’s Amazon rainforest burned in 2023—an area comparable in size to the U.S. state of Tennessee—according to MapBiomas Fire Monitor . This represents a 35.4% increase from the previous year.
On average close to 1 million trees are still being chopped down or burned every day in the Amazon. Countless more died because of the drought, and this will worsen the degradation of the forest. Overall, the Amazon was worse off in 2023 than the year prior.
The Impact of Climate Change, El Niño and Unprecedented Drought:
In 2023, researchers’ forecasts came to fruition as the peak of the Amazon fire season aligned with an ongoing El Niño event—a climate phenomenon characterized by the warming of sea surface temperatures in the equatorial Pacific Ocean, which affects rainfall patterns in the Amazon. This perilous combination led to drier conditions, thereby intensifying forest fires.
Indeed, in 2023, the Amazon experienced the most severe drought in its recorded history , with rivers dropping to record lows, adversely affecting communities and endangering wildlife.
While El Niño significantly contributed to the reduction in rainfall, thereby exacerbating the 2023 drought, preliminary scientific analyses have determined that the drought’s extreme severity was mainly due to high temperatures associated with climate change. The probability of such an extreme drought occurring was increased thirtyfold by the climate crisis.
This convergence of climate change, unprecedented temperatures, extreme drought, deforestation, and wildfires underscores the growing concerns for the Amazon’s future.
Amazon fires made global headlines in 2019—four years later were they as bad?
Unfortunately, they were.
In August 2019, massive smoke plumes drifted thousands of miles from the burning Amazon and settled over Sao Paulo, Brazil’s largest city, blocking out the sun. The world’s attention focused on Jair Bolsonaro’s administration as he opened the Amazon for further exploitation by incentivizing land invasions, mining, ranching and large-scale agriculture, the primary drivers of tropical forest fires.
Despite international pressures, the situation has continued to deteriorate.
Deforestation in the Brazilian Amazon fell by 50% in 2023 compared to the previous year, showing that President Lula da Silva’s efforts to restore environmental protections dismantled by Bolsonaro have achieved some positive results. However, Lula’s administration—weakened in the face of a conservative Congress—has been unable to prevent the passage of legislation that continues to dismantle environmental safeguards and Indigenous peoples’ rights in favor of agribusiness interests. The future of the Amazon hangs in the balance.
Area Burned in Brazil’s Amazon Annually Since 2019
Why Does the Rainforest Burn?
Tropical forests such as the Amazon are very humid, and under natural conditions they rarely burn—unlike many forests in the western United States where fire is a natural part of the forest’s life cycle.
In the last few decades, two interrelated phenomena have driven Amazon fires: drought, and the expansion of industrial agriculture.
Forests cleared for cattle ranching, agriculture, logging, or illegal mining are cut and then deliberately set on fire once the felled trees are dry enough to burn. Typically, the surrounding forest is wet enough to stop the fire at the edges of new fields and pastures. But prolonged drought in the Amazon basin—linked to climate change and deforestation—means fires are escaping into neighboring intact forests and burning out of control across thousands of acres.
As more forests are cleared, new roads are built, more people move into the area, and new fields are cleared for cattle and crops, creating a vicious cycle that both intensifies the drought and exposes more forests to fire threats.
Scientists warn this cycle is leading the entire Amazon towards a ‘tipping point,’ believed to occur when the combined effects of deforestation and degradation surpass a threshold of 20% to 25% . A new study warned that the Amazon rainforest could reach this ‘tipping point’ by as soon as 2050. At that point, the world’s largest tropical forest would become so fragmented that it would no longer retain sufficient moisture to sustain itself, leading to catastrophic consequences for the global climate and life on Earth.
Related News
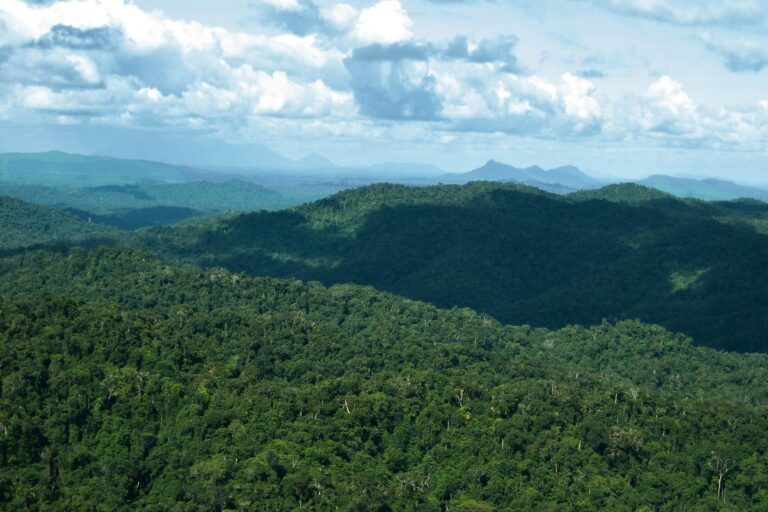
Deforestation in Brazil’s Amazonian Indigenous lands decreased by 42%, reaching a six-year low
Indigenous peoples’ lands in the Amazon experienced a 42% decrease in deforestation between August 2023 and March 2024, according to a report by the Brazilian organization Amazon Institute of People and the Environment (Imazon). This is the lowest amount of destruction recorded within these territories since 2018.
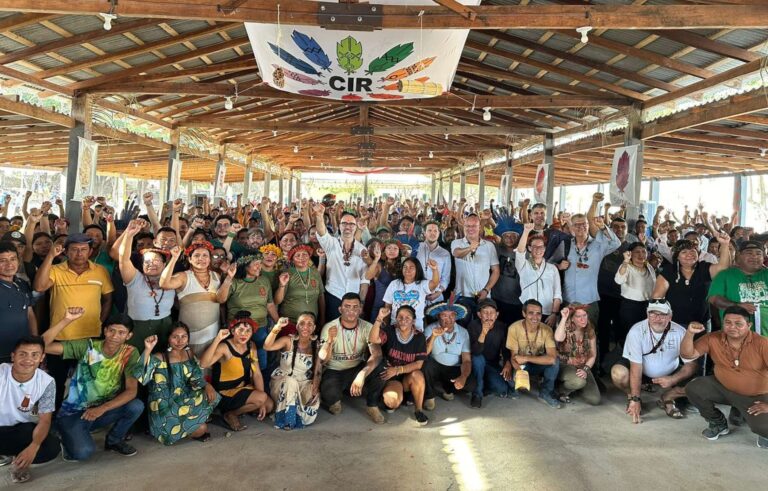
RFUS Joins Indigenous Leaders to Address Climate Challenges and Community Rights in Roraima, Brazil
We have expanded our programming in Brazil to focus on territorial defense, Indigenous governance, and territorial control through community-led forest patrolling. Learn more about our recent visit to Brazil, where we strengthened ties with partners in Roraima, including the Indigenous Council of Roraima’s (CIR), Hutukara – Yanomami Association, Seduume, and Wai-Wai organizations.
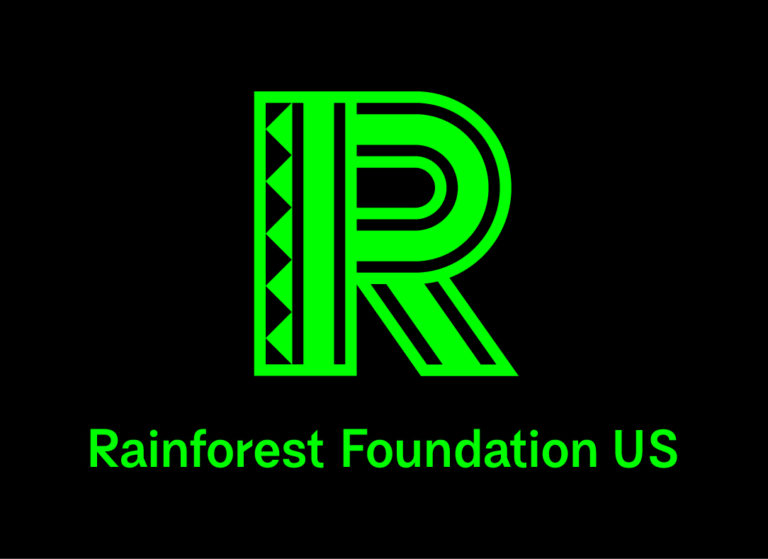
Justice Prevails: Peru Court Sentences Murderers of Indigenous Land Defenders to 28 Years
After ten long years, justice was served on Thursday, April 11, for the victims of the emblematic Saweto case in the Ucayali region of Peru. The Court sentenced the five accused to 28 years and three months of imprisonment for the crimes against Ashéninka community leaders from Alto Tamaya – Saweto: Edwin Chota Valera, Jorge Ríos Pérez, Francisco Pinedo Ramírez, and Leoncio Quintisima Meléndez, who were brutally murdered on September 1, 2014.
Support Our Work
Rainforest Foundation US is tackling the major challenges of our day: deforestation, the climate crisis, and human rights violations. Your donation moves us one step closer to creating a more sustainable and just future.
THE EARTH IS SPEAKING
Will you listen?
Now, through April 30th, your impact will be doubled. A generous donor has committed to matching all donations up to $15,000.
Any amount makes a difference.
Donate Today
Sign up today.
Get updates on our recent work and victories, stories from our Indigenous partners, and learn how you can get involved.

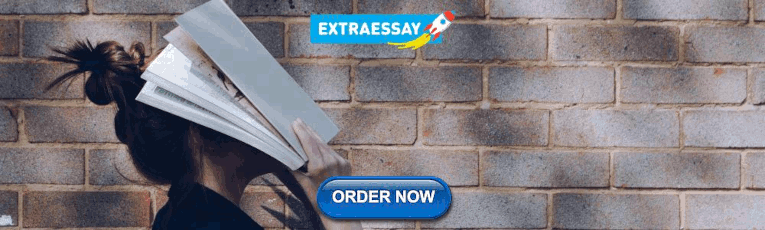
COMMENTS
On a brisk November morning in 2018, a fire sparked in a remote stretch of canyon in Butte County, California, a region nestled against the western slopes of the Sierra Nevada mountains. Fueled by ...
Case study landscapes were approximately 200,000 ha (Table 1), intended to align with the upper limit of the size distribution of wildfires in local ecosystems (During the 2019-2020 fire season ...
David Dee Delgado/Getty Images. New research shows that the health consequences of wildfire smoke exposure stretch well beyond the smoky days themselves, contributing to nearly 16,000 deaths each ...
Here we study the contribution of growing wildfire activity to recent trends in concentrations of ambient particulate matter with diameters less than 2.5 μm (PM 2.5).Wildfires have increased in ...
In particular, recent wildfire case studies suggest that individuals vary in their knowledge and beliefs about their own exposures and about the risks that these exposures pose to their health 19,20.
This is consistent with other studies: ridging is a feature of North America fire spread events 90 and increased wildfire potential 91,92,93,94, while a long-lived block was implicated in the 2010 ...
The world has already observed many devastating effects of human-induced climate change. 1 A vivid manifestation is the several large wildfires that have occurred recently — in some cases, fires ...
1 Introduction. Wildfire activity in the United States (U.S.) and across the globe has increased markedly over the last several decades (e.g., Balch et al., 2017; Dennison et al., 2014; Iglesias et al., 2022; Westerling et al., 2006).In the U.S., many of the recent wildfire seasons have involved long and intense burning periods, leading to the loss of life and property, as well as poor air ...
The Camp Fire ignited on November 8, 2018 in the foothills of the Sierra Nevada in Butte County, California. The first 24 hours were characterized by a fast-moving fire with initial spread driven by high winds up to 22 m/s (50 mi/h) and long-range spotting up to 6.3 km (3.9 mi) into the community. The fire quickly impacted the communities of ...
Global warming driving California wildfire trends - study. Climate change is driving the scale and impact of recent wildfires that have raged in California, say scientists. Their analysis finds an ...
Background California in the year 2020 experienced a record breaking number of large fires. Here, we place this and other recent years in a historical context by examining records of large fire events in the state back to 1860. Since drought is commonly associated with large fire events, we investigated the relationship of large fire events to droughts over this 160 years period. Results This ...
All landscapes are forested, have considerable Wildland Urban Interface (WUI), and have a history of both wildfire and prescribed fire. Case study landscapes were approximately 200,000 ha (Table (Table1), 1), intended to align with the upper limit of the size distribution of wildfires in local ecosystems (During the 2019-2020 fire season the ...
"Tragically, we are releasing these reports in the aftermath of the fire on Maui Island in Hawaiʻi. Our thoughts are with everyone affected by this terrible event." The Camp Fire Case Study Report. The Camp Fire began in Northern California's Butte County on the morning of Nov. 8, 2018.
The Camp Fire, California's most destructive wildfire in history, burned in Butte County from November 8 through November 25, 2018, claiming at least 86 lives and causing an estimated $16.5 billion in damage. It burned more than 18,000 buildings, including the majority of the town of Paradise.
Canada's 2023 wildfire season is the most destructive ever recorded, and it's not over yet. By September 5, more than 6,132 fires had torched a staggering 16.5 million hectares of land. To put that in perspective, that's an area larger than Greece and more than double the 1989 record.
Tragically, 85 people lost their lives in the fire, making it the deadliest wildfire in U.S. history in the past 100 years. Pulga (★) is a rural community in Butte County, California. It is located along the west slope of the Feather River canyon, at an elevation of 1,398 feet. The distance from Pulga (★) to Paradise ( ) is about 12 miles ...
The fire also released a large amount of CO2 and other toxic contaminants including heavy metals and PAHs deposited on trees and soils. ... The recent dry climate is the main cause of the severity of this explosive wildfire. Tree roots and soils were scorched. ... Those interested in contributing to this case study may use the following ...
In an analysis of satellite images, the authors detected a rise in forest wildfires from 13,477 in 2022 to 34,012 in 2023. The main cause was drought. The region has been experiencing longer and ...
Wildfires, made more severe by climate change, threaten areas beyond those in the West that have recently experienced catastrophic fires. This increasing exposure to wildfire risk puts utility finances in jeopardy and, in turn, impedes the construction of electric infrastructure needed for a transition to renewable energy.
Case Study: Climate Change and the Risk of Wildfires in Canada The year 2021 was one of Canada's busiest fire seasons in recent history according to Natural Resources Canada. The fire season started early with dry spring conditions in the prairies. From March to May, communities in Alberta, Saskatchewan and Manitoba received evacuation alerts ...
Wildfire smoke in the U.S. had a "notable influence" on recent air quality trends in nearly 75% of states in the continental U.S., despite air quality that has been steadily improving over the ...
Abstract. The August 8, 2023R Lahaina fire refocused attention on wildfires, public alerts, and emergency management. Wildfire risk is on the rise, precipitated through a combination of climate change, increased development in the wildland-urban interface (WUI), decades of unmitigated biomass accumulation in forests, and a long history of emphasis on fire suppression over hazard mitigation.
The Wildland Fire Lessons Learned Center (LLC) website serves as a hub of resources to facilitate learning across the interagency wildland fire community. Wildland Firefighters from federal, state, tribal, and local agencies visit the site seeking lessons, statistics, analyses, and to support organizational learning at all levels. The LLC staff works to meet the field where they are with ...
In the province of British Columbia, Canada, four of the most severe wildfire seasons of the last century occurred in the past 7 years: 2017, 2018, 2021, and 2023. To investigate trends in ...
In a recent Los Angeles Times interview, U.S. National Park Service Director Jon Jarvis, said about the Rim Fire, "It is a fire that's ... CoreLogic Wildfire Case Studies Subject: A high-level wildfire analysis of the Yarnell Hill Fire in Arizona, the Black Forest Fire in Colorado, and the Rim Fire in California in 2013. ...
A staggering 26.4 million acres of Brazil's Amazon burned in 2023, a 35.4% increase from the previous year. Deforestation from agriculture and cattle ranching is a main driver of forest fires in the Amazon. Despite recent changes in Brazil's leadership and President Lula's pledges to protect the Amazon, many powerful business interests and politicians continue to put profit over people and ...
The start of the Hayman Fire was timed and located perfectly to take advantage of these conditions resulting in a wildfire run in 1 day of over 60,000 acres and finally impacting over 138,000 acres. The Hayman Fire Case Study, involving more than 60 scientists and professionals from throughout the United States, examined how the fire behaved ...
Citing a recent study, t he researchers said carbon emissions from high-intensity fires — those that occur when weather conditions are hot, dry, and windy — have more than doubled since 2000.
Australian wildfire case study wildfires, australia areas affected causes impacts responses more than million hectares of land were burned. regions across the. ... Aus wildfire case study recent. Australian wildfire case study. Subject. Physical Geography. 405 Documents. Students shared 405 documents in this course. Degree Sixth Form (A Levels)
Case study: wildfires. One of the major predictions made about climate change is that incidents of extreme weather will become more common. This can include weather events such as drought ...