Methicillin-resistant Staphylococcus aureus: an overview of basic and clinical research
Affiliations.
- 1 Duke University Medical Center, Durham, NC, USA.
- 2 Division of Infectious Diseases, Fondazione IRCCS Ca' Granda Ospedale Maggiore Policlinico, Milan, Italy.
- 3 Duke University Medical Center, Durham, NC, USA. [email protected].
- 4 Duke Clinical Research Institute, Durham, NC, USA. [email protected].
- PMID: 30737488
- PMCID: PMC6939889
- DOI: 10.1038/s41579-018-0147-4
Methicillin-resistant Staphylococcus aureus (MRSA) is one of the most successful modern pathogens. The same organism that lives as a commensal and is transmitted in both health-care and community settings is also a leading cause of bacteraemia, endocarditis, skin and soft tissue infections, bone and joint infections and hospital-acquired infections. Genetically diverse, the epidemiology of MRSA is primarily characterized by the serial emergence of epidemic strains. Although its incidence has recently declined in some regions, MRSA still poses a formidable clinical threat, with persistently high morbidity and mortality. Successful treatment remains challenging and requires the evaluation of both novel antimicrobials and adjunctive aspects of care, such as infectious disease consultation, echocardiography and source control. In this Review, we provide an overview of basic and clinical MRSA research and summarize the expansive body of literature on the epidemiology, transmission, genetic diversity, evolution, surveillance and treatment of MRSA.
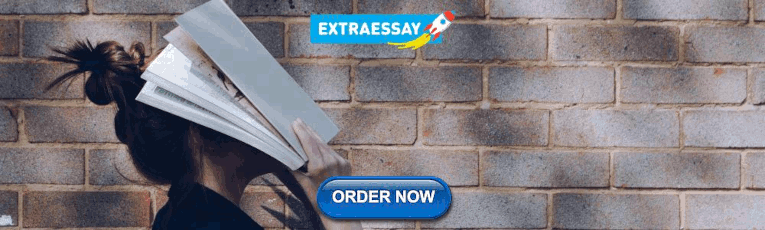
Publication types
- Research Support, N.I.H., Extramural
- Anti-Bacterial Agents / pharmacology*
- Bacteremia / epidemiology
- Clinical Trials as Topic
- Community-Acquired Infections / epidemiology
- Community-Acquired Infections / microbiology
- Cross Infection / epidemiology
- Cross Infection / microbiology
- Evolution, Molecular
- Genetic Variation
- Methicillin / pharmacology*
- Methicillin-Resistant Staphylococcus aureus / drug effects*
- Methicillin-Resistant Staphylococcus aureus / genetics*
- Methicillin-Resistant Staphylococcus aureus / pathogenicity
- Soft Tissue Infections / epidemiology
- Soft Tissue Infections / microbiology
- Staphylococcal Infections / drug therapy
- Staphylococcal Infections / epidemiology*
- Anti-Bacterial Agents
- Methicillin
Grants and funding
- K24 AI093969/AI/NIAID NIH HHS/United States
- UM1 AI104681/AI/NIAID NIH HHS/United States
- S10 OD018164/OD/NIH HHS/United States
- U01 AI124319/AI/NIAID NIH HHS/United States
- R01 AI068804/AI/NIAID NIH HHS/United States
- KL2 TR002554/TR/NCATS NIH HHS/United States
Thank you for visiting nature.com. You are using a browser version with limited support for CSS. To obtain the best experience, we recommend you use a more up to date browser (or turn off compatibility mode in Internet Explorer). In the meantime, to ensure continued support, we are displaying the site without styles and JavaScript.
- View all journals
- Explore content
- About the journal
- Publish with us
- Sign up for alerts
- Published: 31 May 2018
Methicillin-resistant Staphylococcus aureus
- Andie S. Lee 1 , 2 ,
- Hermínia de Lencastre 3 , 4 ,
- Javier Garau 5 ,
- Jan Kluytmans 6 , 7 ,
- Surbhi Malhotra-Kumar 8 ,
- Andreas Peschel 9 , 10 &
- Stephan Harbarth 11
Nature Reviews Disease Primers volume 4 , Article number: 18033 ( 2018 ) Cite this article
114k Accesses
775 Citations
483 Altmetric
Metrics details
- Antibacterial drug resistance
- Bacterial infection
- Disease prevention
Since the 1960s, methicillin-resistant Staphylococcus aureus (MRSA) has emerged, disseminated globally and become a leading cause of bacterial infections in both health-care and community settings. However, there is marked geographical variation in MRSA burden owing to several factors, including differences in local infection control practices and pathogen-specific characteristics of the circulating clones. Different MRSA clones have resulted from the independent acquisition of staphylococcal cassette chromosome mec (SCC mec ), which contains genes encoding proteins that render the bacterium resistant to most β-lactam antibiotics (such as methicillin), by several S. aureus clones. The success of MRSA is a consequence of the extensive arsenal of virulence factors produced by S. aureus combined with β-lactam resistance and, for most clones, resistance to other antibiotic classes. Clinical manifestations of MRSA range from asymptomatic colonization of the nasal mucosa to mild skin and soft tissue infections to fulminant invasive disease with high mortality. Although treatment options for MRSA are limited, several new antimicrobials are under development. An understanding of colonization dynamics, routes of transmission, risk factors for progression to infection and conditions that promote the emergence of resistance will enable optimization of strategies to effectively control MRSA. Vaccine candidates are also under development and could become an effective prevention measure.
Similar content being viewed by others
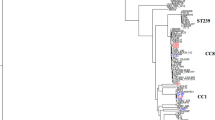
Genetic and phenotypic diversity of methicillin-resistant Staphylococcus aureus among Japanese inpatients in the early 1980s
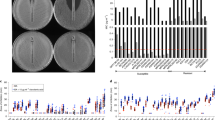
Genomic identification of cryptic susceptibility to penicillins and β-lactamase inhibitors in methicillin-resistant Staphylococcus aureus
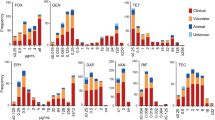
Known mechanisms cannot account for a third of reduced susceptibility in non-aureus staphylococci
Introduction.
Staphylococcus aureus is a Gram-positive, nonmotile, coagulase-positive coccoid bacterium of the Firmicutes phylum. Although the Staphylococcus genus includes 52 species and 28 subspecies ( List of Prokaryotic names with Standing in Nomenclature ), S. aureus is by far the most clinically relevant. S. aureus is found in the human commensal microbiota of the nasal mucosa in 20–40% of the general population 1 , 2 . The reported prevalence varies owing to differences in the size and demographics of the study populations, quality of sampling and culture techniques utilized 3 . When the cutaneous and mucosal barriers are disrupted, for example, owing to chronic skin conditions, wounds or surgical intervention, S. aureus can gain access to the underlying tissues or the bloodstream and cause infection. Persons with invasive medical devices (such as peripheral and central venous catheters) or compromised immune systems are particularly vulnerable to S. aureus infection 4 .
Methicillin-resistant S. aureus (MRSA) was first described in England in 1961 (Ref. 5 ), soon after methicillin was introduced into clinical practice. Methicillin was initially widely used; however, because of its toxicity, it is now no longer marketed for human use and has largely been replaced by similar, more-stable penicillins such as oxacillin, flucloxacillin and dicloxacillin 6 . Nevertheless, the term methicillin-resistant S. aureus continues to be used. In the decade following its initial description, MRSA was responsible for hospital outbreaks (health-care-associated MRSA (HA-MRSA)) in many parts of the world 7 . A substantial change in MRSA epidemiology was observed when it was detected in individuals without previous health-care contact (referred to as community-associated MRSA (CA-MRSA)), notably among indigenous populations in Australia in the 1980s 8 and otherwise healthy persons, including children, in the United States in the 1990s 9 . Since the mid-2000s, it has also been associated with livestock exposure (livestock-associated MRSA (LA-MRSA)) 10 .
Several S. aureus clones (that is, bacteria that are indistinguishable from each other by a variety of genetic tests (for example, pulsed-field gel electrophoresis, multilocus enzyme electrophoresis or ribotyping) or that are so similar that they are presumed to be derived from a common parent 11 ) have developed into MRSA by uptake via horizontal gene transfer of staphylococcal cassette chromosome mec (SCC mec ) 12 , a mobile genetic element that encodes the genes mecA or mecC , which confer resistance to methicillin and, therefore, to most β-lactam antibiotics. MRSA is often also resistant to multiple other antibiotic classes. Indeed, S. aureus has the remarkable ability to acquire resistance to any antibiotic 7 , which has major implications for current as well as future treatment options for this pathogen.
Individuals with MRSA colonization or carriage (that is, the presence of bacteria that do not cause a detectable host immune response, cellular damage or clinical signs and symptoms of infection) have an increased risk of subsequent infection and are an important source of person-to-person transmission. Health-care facilities host persons who are predisposed to infection (for example, owing to invasive procedures and/or immune compromise) and are environments with high antibiotic selection pressure (which can contribute to the selection of antimicrobial resistance in bacteria) and frequent contact between individuals. These conditions have facilitated the epidemic spread of MRSA in hospitals; MRSA is now endemic in many health-care facilities throughout the world and, as a consequence, it has become a major focus for infection control efforts globally.
This Primer reviews the epidemiology, pathophysiology, diagnosis, prevention, management and clinical effect of MRSA, focusing on HA-MRSA, and discusses future research priorities. In some aspects of the epidemiology and pathophysiology, where methicillin resistance does not substantially affect the behaviour of the species, data regarding S. aureus in general have also been included.
Epidemiology
The emergence and worldwide spread of MRSA represent some of the most important events in the epidemiology of infectious diseases. Although MRSA was first reported in the early 1960s 5 , whole-genome sequencing (WGS) of 209 early MRSA isolates suggests that MRSA emerged in the mid-1940s — that is, much earlier than the introduction of methicillin 13 . In fact, it has been hypothesized that it was the extensive use of penicillin rather than the introduction of methicillin that drove the emergence of MRSA 13 .
Many countries have experienced an increasing burden of MRSA since the 1960s. The burden of MRSA has notable geographical variation, ranging from low prevalence in Scandinavia to the highest prevalence in parts of America and Asia 14 ( Fig. 1 ). The spread of MRSA seems to occur by at least two mechanisms: spread of existing resistant clones and acquisition of SCC mec by a methicillin-sensitive S. aureus (MSSA) strain (a strain is a descriptive subdivision of a species based on phenotypic and/or genotypic characteristics 11 ). Details of the mechanism of horizontal transfer of SCC mec are not well understood, but epidemiological evidence shows that this resistance mechanism has spread to most clones of S. aureus , in both human and animal pathogenic strains 15 , 16 . The following sections discuss the epidemiology of MRSA in different regions; in general, less comprehensive data are available from low-income and middle-income countries.
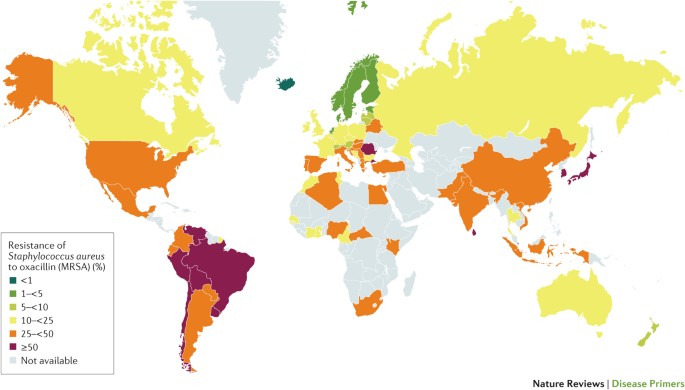
The percentage of Staphylococcus aureus isolates that are resistant to oxacillin (that is, methicillin-resistant S. aureus (MRSA) isolates) is shown. Data include aggregated resistance rates. Owing to differences in the scope of collections and testing methods, caution should be exercised in comparing data across countries. Data represented are adapted from the Center for Disease Dynamics, Economics & Policy Resistance Map ; data for the following countries are adapted from Ref. 223 , Elsevier: Algeria, Bolivia, Brazil, Cameroon, Central African Republic, Chile, Colombia, Egypt, Hong Kong, Indonesia, Ivory Coast, Japan, Kenya, Malta, Morocco, Nigeria, Paraguay, Peru, Senegal, Singapore, South Korea, Sri Lanka, Tunisia and Uruguay.
PowerPoint slide
MRSA in Europe
Surveillance data from European countries show a general trend towards increasing MRSA prevalence from the north to the south of the continent, with <5% of S. aureus isolated from invasive infections being methicillin-resistant in northern Europe (for example, the Netherlands, Norway, Sweden and Denmark) compared with 25–50% in southern Europe (for example, Portugal, Spain, Italy and Greece) 17 ( Fig. 2 ). Varying infection control practices and antimicrobial usage are thought to contribute to the observed differences 18 .
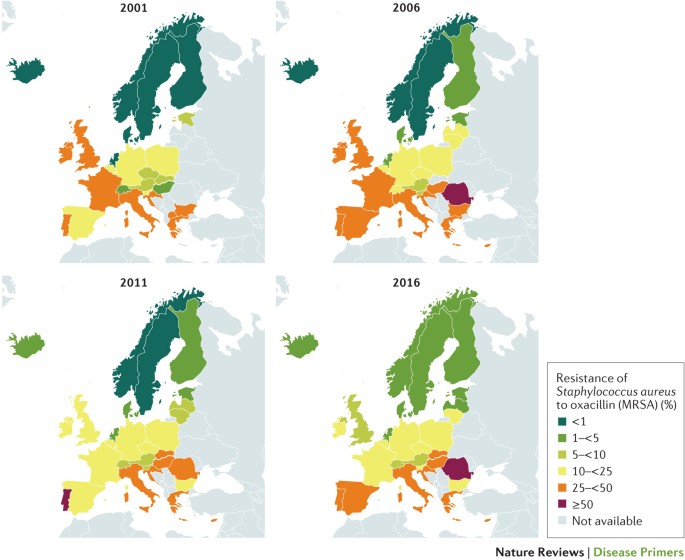
Surveillance data show that methicillin-resistant Staphylococcus aureus (MRSA) prevalence generally increases from the north to the south of Europe. Between 2001 and 2016, several European countries reported a decreasing trend in the prevalence of MRSA. For example, France and the United Kingdom have experienced declining MRSA rates since the early 2000s, which are largely attributed to improved multifaceted national infection control programmes 224 , 225 . Figure adapted with permission from Surveillance Atlas of Infectious Diseases , European Centre for Disease Prevention and Control.
After years of increasing MRSA prevalence, since the early 2000s, steady or decreasing prevalence has been observed in a number of countries 17 ( Fig. 2 ). This decline has been associated with the implementation of improved national control interventions. However, some experts argue that widespread declines in previously hyperendemic MRSA clones are attributable to changes in the organism itself, with loss in survival fitness resulting in shifts in circulating clones 19 , 20 .
With the declines largely occurring in HA-MRSA, there has been increasing recognition of animal reservoirs for human MRSA in Europe, particularly from food-producing animals such as pigs, cattle and poultry 21 . This LA-MRSA, predominantly belonging to clonal complex 398 (CC398), has primarily caused infections in those who work with livestock (particularly in the Netherlands, northwestern Germany 22 and Spain 23 ), but LA-MRSA infections have also been observed among the general population 24 , 25 . However, at present, sustained person-to-person transmission of LA-MRSA seems to be uncommon 25 , 26 .
MRSA in America
In the United States, ∼ 53% of S. aureus clinical isolates were methicillin-resistant in 2005 (Ref. 27 ). MRSA has also been identified as the most common cause of skin and soft tissue infections (SSTIs) presenting to US hospital emergency departments, which is largely attributed to the emergence of CA-MRSA (particularly the USA300 clone in the early 2000s) 28 , 29 ( Fig. 3 ). The changes in epidemiology since the emergence of CA-MRSA in the country have made the distinction between CA-MRSA and HA-MRSA less clear 30 , 31 . A parallel epidemic of CA-MRSA closely related to USA300, the USA300 Latin-American variant (USA300-LV), was first identified in Colombia in 2005 and has emerged as the most prevalent CA-MRSA clone in northern South America 32 . The geographical spread of USA300 clones from South America to Europe has also been documented by genomic analysis of MRSA isolates in Switzerland 33 .
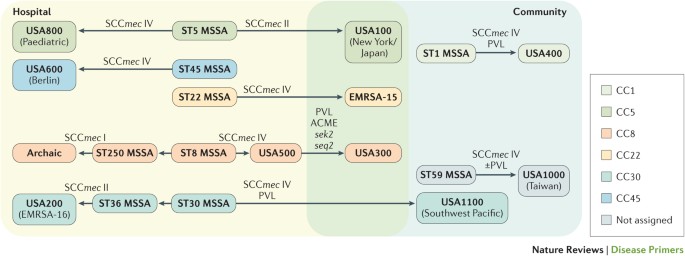
Sequence types (STs) of methicillin-sensitive Staphylococcus aureus (MSSA) are grouped into clonal complexes (CCs) by their similarity to a founder allelic profile (genotype) 226 . STs have different molecular properties that enable monitoring of the geographical spread of different clones. STs of MSSA can evolve into MRSA by acquiring staphylococcal cassette chromosome mec (SCC mec ), of which there are different types (represented by roman numerals). Commonly used clone names are within parentheses. Numbers in the names of MRSA USA clones are based on pulsed-field gel electrophoresis analysis. ACME, arginine catabolic mobile element; EMRSA, epidemic MRSA; PVL, Panton–Valentine leukocidin; sek2 and seq2 are staphylococcal genes encoding enterotoxins. Figure adapted with permission from Ref. 227 , Oxford University Press.
Similar to experiences in Europe, the incidence of HA-MRSA in the United States has shown decreasing trends since 2005, with hospital-onset HA-MRSA infections decreasing by 54% 34 . In 2007, Veterans Affairs Hospitals throughout the United States introduced a multifaceted prevention strategy including universal MRSA screening, contact precautions, hand hygiene promotion and institutional culture change 35 . This programme was associated with a significant reduction in MRSA infections by 62% in intensive care units (ICUs) and 45% in other hospital wards. In addition, many US states mandated specific MRSA control measures, and in 2008, the Centers for Medicare and Medicaid Services introduced financial penalties to hospitals for preventable health-care-acquired infections, although a large evaluation of this intervention showed no evidence that it was associated with a reduction in health-care-associated infections 36 .
MRSA in the Asia–Pacific region
MRSA is endemic in most hospitals in Asia, and some Asian countries have among the highest MRSA prevalence in the world 37 . However, most available data are from high-income countries (for example, Japan, South Korea and Singapore), with limited information from other nations. Although there is country-to-country variability, MRSA accounts for up to 50% of S. aureus bloodstream infections in parts of Asia 37 . Japan and South Korea have particularly high MRSA prevalence — with >70% of clinical isolates in South Korea being MRSA on the basis of regional surveillance data from 2011 (Refs 37 , 38 ). High methicillin resistance rates are thought to be related to widespread inappropriate antimicrobial use (for example, self-medication and over-the-counter use) as well as high population density facilitating rapid transmission of multidrug-resistant organisms 37 . Nevertheless, some countries in Asia (for example, Taiwan 39 ) that experienced a peak in HA-MRSA prevalence in the late 1990s have shown declining prevalence since the early 2000s 38 .
In Australia, among health-care-associated S. aureus , MRSA has accounted for 20–33% of isolates since 2001 (Ref. 40 ). Like Europe and the United States, Australia has implemented a range of local and national interventions that have been associated with a reduction in HA-MRSA bloodstream infections since 2002 (Ref. 41 ). Since the earliest reports of CA-MRSA in remote indigenous populations in Western Australia in the late 1980s 8 , several distinct CA-MRSA clones circulating in the region have been identified, including virulent clones such as sequence type 93 (ST93; Queensland clone) and ST30 (Southwest Pacific clone) 42 .
MRSA in Africa
MRSA prevalence data from Africa are variable in coverage and quality. Published data are available for South Africa, Nigeria and countries from the Mediterranean basin, but there is a paucity of data from other nations 43 . Most data are also from single-centre studies, and information from broader surveillance systems is lacking. In addition, most studies have relied on phenotypic methods to identify MRSA, and these tests might be less reliable than genotypic methods depending on the choice of antibiotic used to detect MRSA 43 .
MRSA prevalence is estimated at <50% in most countries, with several countries reporting prevalence of <25% 43 . However, MRSA prevalence has been increasing since the early 2000s in reports from most countries, although it has started to decrease in South Africa (from 36% in 2006 (Ref. 44 ) to 24% during 2007–2011 (Ref. 45 )) 43 . Differences in the availability and use of antimicrobials, incidence of HIV infection (a risk factor for MRSA colonization 46 ) and infection control practices could potentially account for some of the variation between countries.
Mechanisms/pathophysiology
Staphylococcus aureus colonization.
S. aureus colonization precedes the development of infection in most cases 47 . Less commonly, infection can occur in the absence of known S. aureus colonization, for example, as a result of contamination of catheters or wounds owing to suboptimal infection control practices by health-care workers. The principal site of S. aureus colonization is the nose, although colonization at other sites occurs, notably in the throat and perineum 48 . Longitudinal studies have identified three temporal patterns of S. aureus (including both MSSA and MRSA) colonization 49 . Continuous S. aureus colonization was found in ∼ 15% of individuals (known as persistent carriers), intermittent colonization was present in 70% of individuals (which means that the majority of individuals can repeatedly acquire S. aureus and spontaneously clear it), and S. aureus was never detected in 15% of individuals (referred to as non-carriers) 49 . Similar results were found by other studies 50 . Studies exploring specific host polymorphisms in genes involved in the inflammatory response 51 indicate that there are underlying host factors that determine the carriage status. However, the precise nature of these underlying factors is not completely understood. For MRSA in particular, the duration of colonization is variable, and reported estimates could be biased by antibiotic treatment, which can shorten the duration of colonization. In one study in patients with MRSA colonization at the time of hospital discharge 52 , the median duration of colonization was 282 days; in this population, 81% of individuals had chronic skin lesions, a known risk factor for MRSA colonization, which may have contributed to prolonged carriage. Besides host factors, factors associated with the pathogen itself as well as the nasal microbiota can influence host carrier status.
Dynamics of colonization. During S. aureus colonization, initial bacterial adherence to the host's epithelial cells is mediated by teichoic acid on the cell wall, whereas microbial surface components recognizing adhesive matrix molecules play a part at a later stage of nasal colonization 53 , 54 . Of these components, S. aureus clumping factor B (ClfB), has been studied in vitro and in human volunteers 55 . A wild-type strain and its single locus clfB knockout variant were inoculated into the nose; the knockout variant was cleared significantly more rapidly than the wild-type strain. However, ClfB-deficient strains can still interact with nasal cells, indicating that there are several independent microbial surface components that play a part in colonization 56 . It must also be noted that only one strain was used in this study.
Besides host and pathogen factors, the interaction of S. aureus with other nasal-colonizing species (for example, Corynebacterium spp., Propionibacterium acnes , Staphylococcus lugdunensis and Staphylococcus epidermidis ) has a role in S. aureus colonization. Studies of the nasal microbiota have shown that the presence of some species correlates with the presence or absence of S. aureus (for example, S. epidermidis has been positively correlated with the presence of S. aureus ) 57 , 58 . The organisms of the nasal microbiota are in competition with each other in several ways. For example, they compete for adhesion sites and nutrients: there are low amounts of nutrients in the human nose. S. aureus can survive in environments with lower levels of nutrients than coagulase-negative staphylococci can 59 , possibly owing to differences in metabolism, and hence is better adapted to the human nose. However, no difference in nutrient levels has been observed between carriers and non-carriers 59 . Microbiota species also compete by antibiosis, that is, certain strains can produce antimicrobial molecules that inhibit their microbial competitors. S. lugdunensis , for example, produces an antimicrobial compound called lugdunin that inhibits and destroys S. aureus (including MRSA) in vitro and in a mouse model, possibly by leading to rapid breakdown of bacterial energy resources 60 . In humans, nasal colonization with S. lugdunensis has been associated with a sixfold lower risk of colonization with S. aureus . These findings are certainly interesting but explain only a minority of carriage patterns, as S. lugdunensis colonization has been reported in only 9 – 26% of the general population 60 , 61 . Finally, S. aureus also competes by induction of host defences, that is, it induces the production of host antimicrobial proteins that are less harmful to S. aureus than to other commensal bacteria 62 . Many studies support the role of these mechanisms in the interactions between S. aureus and the commensal microbiota, but a single mechanism is insufficient to explain all observed carriage patterns.
S. aureus has an extensive arsenal of virulence factors (including adhesive, host-cell damaging and immunomodulatory molecules) that vary in their presence or specificity between clones 63 , 64 , a variability that is reflected by the high diversity of infections that S. aureus can cause 65 , 66 ( Table 1 ). Many virulence genes are found on mobile genetic elements; thus, their combination differs substantially between clones and even between closely related strains. The potential association of specific virulence factors with certain types or aggressiveness of S. aureus infections remains elusive, probably because many of these factors have redundant, partially overlapping functions. Furthermore, many virulence factors cannot be investigated in animal models because they are human-specific 67 . This section focuses on the most prominent virulence mechanisms and typical routes of invasion.
Initiation of infection. S. aureus SSTIs are usually initiated by bacterial transfer (probably via hand contact) from the major reservoir in the nose to open microlesions and wounds on the skin 68 , 69 ( Fig. 4a ). S. aureus surface proteins (for example, fibronectin-binding protein A (FnBPA), FnBPB, clumping factor A (ClfA), ClfB and collagen adhesin (Cna)) bind to extracellular matrix proteins and enable the bacteria to attach to and multiply on wounded tissues 70 . The capacity of S. aureus to adhere to and form biofilms (that is, sticky agglomerations of microorganisms embedded in an extracellular matrix; biofilms facilitate resistance to mechanical interference, host defences and antibiotic treatment) on artificial plastic or metal surfaces renders S. aureus a frequent cause of catheter-associated or joint-replacement-associated infections or of ventilator-associated pneumonia 71 . The subsequent influx of polymorphonuclear leukocytes (PMNs) is manipulated by S. aureus 72 , which shapes local inflammation 73 .
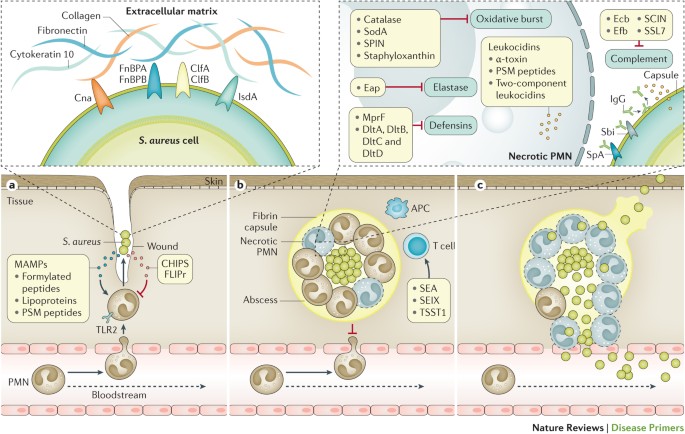
a | Bacteria obtain access to sterile tissues via open wounds and use adhesin proteins, such as fibronectin-binding protein A (FnBPA), FnBPB, iron-regulated surface determinant protein A (IsdA), clumping factor A (ClfA), ClfB and collagen adhesin (Cna), for specific attachment to extracellular matrix proteins, such as fibronectin, cytokeratin 10 and collagen, among others. Staphylococcus aureus can also in part regulate polymorphonuclear leukocyte (PMN) influx in subtle ways involving activators (formylated peptides and phenol-soluble modulin (PSM) peptides) and inhibitors (for example, chemotaxis inhibitory protein of S. aureus (CHIPS) and FPRL1 inhibitory protein (FLIPr)) of PMN chemotaxis 72 . PSM peptides also promote the release of pro-inflammatory lipoproteins, the major S. aureus microorganism-associated molecular pattern (MAMP) molecules, which activate Toll-like receptor 2 (TLR2) and contribute to local inflammation 73 . b | S. aureus produces coagulases to polymerize fibrin and form an encapsulated abscess around the infection site. The capacity of PMNs, which are found in high numbers in an abscess, to eliminate S. aureus is limited by leukocidins and by virulence factors interfering with opsonophagocytosis and PMN killing. S. aureus can compromise effective opsonization by antibodies using a polysaccharide microcapsule and surface proteins ( Staphylococcus protein A (SpA) and immunoglobulin-binding protein Sbi) binding immunoglobulin G (IgG) via the crystallizable fragment (Fc) domain in a futile way 66 . The bacteria can also inhibit the complement signalling pathway by small secreted inhibitors such as staphylococcal complement inhibitor (SCIN), fibrinogen-binding protein (Efb), extracellular complement-binding protein (Ecb) or staphylococcal superantigen-like protein 7 (SSL7), among others. Phagocytosed bacteria can survive within the PMNs by producing catalase, superoxide dismutase [Mn] 1 (SodA), staphylococcal peroxidase inhibitor (SPIN), staphyloxanthin (against the bactericidal oxidative burst generated by the PMNs) 72 and extracellular adherence protein (Eap) (against elastase) 76 , and the cell envelope modifications mediated by multiple peptide resistance factor (MprF) and the D -alanine transfer proteins DltA, DltB, DltC and DltD protect against defensins. S. aureus also secretes cytolytic toxins that can kill PMNs; S. aureus leukocidins include large pore-forming proteins (α-toxin and several two-component leukocidins, such as Panton–Valentine leukocidin (PVL)) 64 and small peptide (PSM peptides) 228 toxins. Superantigen toxins (toxic shock syndrome toxin 1 (TSST1), enterotoxin type A (SEA), staphylococcal enterotoxin-like X (SEIX) and several others) contribute to exuberant inflammation by nonspecific T cell activation. c | Abscesses can release live bacteria to the surface of the skin and/or the bloodstream at later stages; the plasminogen-activating protein staphylokinase might contribute to bacterial dissemination. APC, antigen-presenting cell.
Abscess formation. The S. aureus coagulase proteins cause the formation of a fibrin pseudo-capsule surrounding bacteria and infiltrated PMNs, thereby preventing further leukocyte influx 74 ( Fig. 4b ). S. aureus can impede opsonization, for instance, by production of a polysaccharide microcapsule 66 and inhibition of the complement cascade 75 . However, the microcapsule is absent from important MRSA clones such as USA300 (Ref. 63 ). Bacteria that are phagocytosed by PMNs can survive not only by counteracting PMN killing mechanisms 72 , 76 , 77 but also by gradually destroying them with the help of cytolytic toxins. For example, many CA-MRSA clones produce pore-forming peptide (phenol soluble modulins (PSMs)) and protein toxins (α-toxin (also known as α-haemolysin) and several bi-component leukocidins such as the Panton–Valentine leukocidin (PVL)), which are host species-specific and bind to host leukocyte membranes, leading to the formation of pores and causing lytic cell death 7 , 78 , thereby increasing bacterial virulence. The massive inflammation elicited by activated or necrotic PMNs is further increased by S. aureus superantigen toxins, which bind to the major histocompatibility complex (MHC) class II of antigen-presenting cells and activate a large percentage of T cells nonspecifically, causing systemic hyper-inflammation referred to as ‘cytokine storms’ 79 .
Systemic infection. Abscesses might be disrupted at later stages, releasing pus and live bacteria either towards the skin surface to promote pathogen transmission or towards the bloodstream to cause bacteraemia ( Fig. 4c ). Endovascular S. aureus can adhere to endothelial surfaces and platelets 80 , 81 , and this adhesion can initiate endocarditis, promote the formation of metastatic abscesses or induce bacterial uptake into endothelial cells, where the bacteria are difficult to reach by antibiotics and host defence molecules 82 . The agglutinating activity of coagulases is thought to contribute to systemic blood coagulation, and massive release of microorganism-associated molecular pattern molecules along with superantigen toxin-induced cytokine storms leads to fulminant systemic inflammation, sepsis and multi-organ failure if the endovascular spread of the bacteria cannot be contained 83 .
Regulation and adaptation. Most of the S. aureus virulence factors are differentially regulated by the accessory gene regulator (Agr) quorum-sensing system and other regulatory networks 84 . Many CA-MRSA clones such as USA300 have very active Agr systems, which leads to abundant expression of toxins and corresponds to a high capacity to cause SSTIs and invasive infections even in healthy individuals 85 . By contrast, many HA-MRSA clones contain an additional SCC mec -encoded phenol-soluble modulin (PSM; PSM mec ), whose mRNA dampens Agr expression 86 . Accordingly, Agr is not very active in many HA-MRSA clones, which produce lower amounts of toxins but higher levels of adhesins and often cause bacteraemia via infected catheters or implanted medical devices. High virulence seems to even be detrimental for S. aureus in bacteraemia, with many isolates from bloodstream infections found to bear Agr-inactivating point mutations 87 . Elucidating virulence mechanisms whose inhibition would render S. aureus most vulnerable will be crucial for the development of new preventive and therapeutic strategies against MRSA.
Mechanisms of methicillin resistance
A crucial event in the evolution of S. aureus was the independent acquisition of the SCC mec complex in the early 1960s by several multidrug-resistant strains (resistant to penicillin, streptomycin, tetracycline and erythromycin 88 ), rendering S. aureus resistant to most members of the β-lactam family of antibiotics 5 ( Fig. 3 ). Twelve known SCC mec types (I–XII) have been identified and are classified according to the type of cassette chromosome recombinase ( ccr ) complex and the class of the mec complex ( Table 2 ). Types I, II and III are large SCC mec elements harbouring genes that confer resistance to several antibiotic classes and are primarily found in HA-MRSA 89 . Smaller elements, such as types IV and V SCC mec , are found in CA-MRSA, such as USA300 and USA400, but also in some widespread HA-MRSA clones, such as ST22-MRSA-IV, ST45-MRSA-IV and ST5-MRSA-VI ( Fig. 3 ; Table 2 ). However, over the years, the distinction between the two epidemiological groups (HA-MRSA and CA-MRSA) has become blurred 90 .
All SCC mec types contain mecA (with the exception of type XI, which contains the homologue mecC ), which encodes penicillin-binding protein 2a (PBP2a) 91 , a peptidoglycan transpeptidase. PBP2a has extremely low affinity for most β-lactam antibiotics; in the presence of β-lactam antibiotics that inhibit the function of the four native S. aureus penicillin-binding proteins (PBP1, PBP2, PPB3 and PBP4), PBP2a can take over the transpeptidase function of peptidoglycan biosynthesis ( Fig. 5 ). A variant of mecA , named mecC , was identified in several S. aureus clones from animal and human isolates 92 ; mecC encodes PBP2a LGA , named after the MRSA strain LGA251 from which it was first isolated. The mechanism of the control of β-lactam resistance in strain LGA251 was compared with the resistance mechanism in MRSA strains that carry mecA 93 , 94 ; in the LGA251 strain, the level of methicillin resistance depends on mecC and on genes in the genetic background of the strain. In 2018, plasmid-borne methicillin resistance based on mecB has been identified in S. aureus 95 , but the mechanism of resistance encoded by mecB is yet to be clarified.
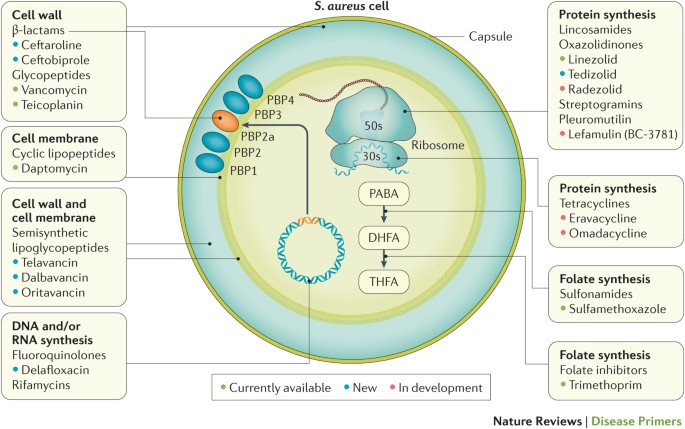
Antibiotics have diverse mechanisms of action and target different bacterial structures or metabolic pathways. Existing antibiotic options are in green, new antibiotics approved and on the market are in blue and antibiotics in the pipeline are in orange. DHFA, dihydrofolic acid; PABA, para-aminobenzoic acid; PBP, penicillin-binding protein; S. aureus , Staphylococcus aureus ; THFA, tetrahydrofolic acid. Figure adapted from Ref. 229 , Macmillan Publishers Limited.
The primary control of the expression of mecA depends on the regulators encoded by mecI , mecR1 and mecR2 (Refs 96 , 97 ) and on the regulators of the expression of the genes blaZ , blaI and blaRI (Ref. 98 ). In addition, a surprisingly large number of genes — auxiliary or fem genes — has a profound influence on the resistant phenotype 99 . Three lines of evidence show that the level of mecA transcription is not predictive of the degree of methicillin resistance. First, the stringent stress response (that is, the bacterial reaction to different stress conditions, such as amino acid, fatty acid and iron limitation and heat shock) induced by the antibiotic mupirocin triggers an increase in PBP2a activity without affecting mecA transcription 100 . Second, inactivation of vraS (a member of the two-component regulatory system involving sensor protein VraS and response regulator protein VraR (VraS–VraR) involved in the control of the cell wall peptidoglycan biosynthesis) induced mecA transcription but did not increase the level of PBP2a activity 101 . Third, the chaperone foldase protein PrsA alters the levels of properly folded PBP2a in the membrane and, therefore, methicillin resistance without affecting mecA transcription 102 . The crucial role of the stringent stress response in mecA expression has been demonstrated using different experimental approaches 99 , 103 . A new line of investigation is focusing on the discovery of inhibitors of the stringent stress response that act in combination with β-lactam antibiotics 103 .
Of note, over the years, some MRSA clones have also acquired resistance to vancomycin 104 , the first-line treatment of invasive MRSA infections in hospitalized patients since the 1960s ( Box 1 ).
Box 1: Vancomycin resistance
Vancomycin has been the drug of choice for treating invasive methicillin-resistant Staphylococcus aureus (MRSA) infections in hospitalized patients since the early 1960s. However, over the years, MRSA has acquired resistance to vancomycin 104 . Vancomycin intermediate-resistant S. aureus (VISA) appeared in Japan in 1997 (Ref. 215 ), and since then, it has been identified worldwide. The VISA phenotype results from mutations acquired during antibiotic therapy 216 . Despite its low vancomycin minimum inhibitory concentration (MIC; the lowest concentration of an antibiotic that prevents bacterial growth) of 3–8 micrograms per millilitre, VISA has been associated with treatment failures 215 . When cultured, heterogeneous VISA (hVISA) strains are phenotypically susceptible to vancomycin but contain subpopulations of VISA colonies at frequencies of 10 −6 to 10 −5 of the cells in the whole population. hVISA seems to be the stage that precedes the development of VISA 217 . The clinical relevance of hVISA has been extensively debated.
Vancomycin failure has been reported for some hVISA or VISA infections; nevertheless, several studies have failed to detect an association between infection with hVISA and poor outcomes with therapy with vancomycin 218 , 219 . For specific cases of hVISA and/or VISA infection, viable alternatives to vancomycin include a combination of high-dose daptomycin with another antibiotic such as gentamicin, rifampin, linezolid, trimethoprim–sulfamethoxazole or a β-lactam. Similarly, if reduced susceptibility to daptomycin is observed alongside reduced vancomycin susceptibility, then a combination of or use of a single agent among the following is recommended: quinupristin–dalfopristin, trimethoprim–sulfamethoxazole, linezolid or telavancin 161 .
Vancomycin-resistant S. aureus (VRSA), which was first detected in the United States in 2002 (Ref. 220 ), has a very high vancomycin MIC (≥32 micrograms per millilitre). Vancomycin resistance in VRSA is mediated by the vanA gene, which is believed to have been transferred from Enterococcus faecalis on the plasmid-borne transposon Tn 1546 (Ref. 221 ). VRSA strains are mostly found in diabetic wounds infected by both vancomycin-resistant enterococci and S. aureus , where there is opportunity for horizontal gene transfer of Tn 1546 harbouring vanA . VRSA has remained extremely rare, possibly owing to the fitness costs associated with acquisition of vancomycin resistance 222 .
Diagnosis, screening and prevention
MRSA can cause a wide range of infections, such as SSTIs, pneumonia, osteoarticular infections, toxic shock syndrome (a rare, potentially life-threatening complication of infection with certain types of bacteria, including S. aureus , caused by the release of bacterial toxins and presenting with clinical features that can include fever, rash and hypotension) and bacteraemia, which may be complicated by endocarditis or severe sepsis 4 . The clinical presentations and risk factors for infection vary between HA-MRSA, CA-MRSA and LA-MRSA strains.
HA-MRSA is a cause of bacteraemia, pneumonia and, less commonly, SSTIs (particularly related to invasive procedures, for example, at surgical wounds or vascular access sites) in hospitalized patients 105 . The organism is often associated with invasive devices, such as intravascular catheters, endotracheal tubes and urinary catheters, probably owing to its capacity to form and survive in biofilms 71 .
Individuals who have had lengthy hospitalization, ICU admission, residency in a nursing home, antibiotic exposure (particularly to cephalosporins and fluoroquinolones, leading to antibiotic selection pressure), surgery, haemodialysis, chronic wounds or indwelling invasive devices have an increased risk of infection with HA-MRSA 106 . In addition, asymptomatic colonization with MRSA is a risk factor for subsequent infection, as individuals with MRSA colonization on admission had a relative risk of infection of 13 (95% CI 2.7–64.0) compared with those with MSSA colonization or 9.5 (95% CI 3.6–25.0) compared with those without S. aureus colonization 107 .
The most common clinical presentation for CA-MRSA is SSTI, which is often associated with abscesses or pus formation and accounts for ∼ 90% of cases 29 . CA-MRSA can cause particularly virulent infections. Fulminant infections with CA-MRSA strains have been reported, such as necrotizing pneumonia and necrotizing fasciitis (a rapidly progressive infection of the fascia with secondary necrosis of the subcutaneous tissues) 108 , 109 . Possible explanations for the increased virulence observed with CA-MRSA strains are very active Agr systems and the production of PVL. However, the presence of PVL varies from strain to strain, suggesting that other virulence factors contribute 7 .
Individuals with CA-MRSA infection usually lack the traditional risk factors associated with HA-MRSA strains. Populations or settings in which outbreaks of CA-MRSA infection have been reported include sports teams, military personnel and prisons 110 , 111 . On the basis of these observations, close contact with MRSA carriers (as occurs in households or other communal living environments), shared equipment or personal items and skin trauma (including trauma caused by injecting drug use or body shaving) might be associated with an increased risk of CA-MRSA infection. However, the distinction between CA-MRSA and HA-MRSA is becoming increasingly blurred, with transmission of CA-MRSA strains now being observed in health-care settings in some countries with high CA-MRSA burden, such as Greece 112 .
LA-MRSA has been associated with localized infections, such as SSTIs (including abscesses and wound infections) and otitis, as well as severe and invasive infections, such as bacteraemia, pneumonia, osteoarticular infections and endocarditis 22 . LA-MRSA predominantly colonizes and infects individuals who have direct contact with livestock (including cattle, horses, chickens and turkeys but particularly pigs) and their household members through transmission within the household 25 . However, there are reports of LA-MRSA in individuals with no connection to livestock, and in these cases, spread via environmental contamination or, less commonly, food-borne transmission has been postulated 25 .
Microbiological diagnosis
Microbiological specimens from which MRSA can be isolated can be broadly classified into clinical and screening samples. Clinical samples (for example, specimens of purulent discharge, deep tissues, sputum and blood) are collected from individuals with symptoms or signs to investigate for active infection, whereas screening samples (for example, nasal, perineal and throat swabs) are obtained to detect asymptomatic colonization. An array of phenotypic and non-phenotypic methods can be used to detect MRSA directly from clinical or screening samples or to identify MRSA from presumptive staphylococcal colonies isolated from clinical samples. Phenotypic methods are usually preferred for clinical diagnostics.
Phenotypic methods. Pure S. aureus cultures, obtained by plating clinical samples on relevant culture media, can be screened for methicillin resistance by the disk-diffusion method. This method involves applying a cefoxitin disk on Mueller-Hinton agar or supplementing Mueller-Hinton agar with 6 micrograms per millilitre oxacillin and 4% NaCl (Clinical and Laboratory Standards Institute (CLSI) recommendations) 113 .
Initially, oxacillin was utilized as the marker antibiotic to detect MRSA; however, CLSI now recommends cefoxitin, as it is a better inducer of mecA and mecC than oxacillin and results in a clear recognizable phenotype 113 . The disk-diffusion method requires strict adherence to temperature (35 °C) and time (reading after 24 hours) to prevent false negative results. This is because the mecA encoded PBP2a is less efficient at crosslinking the pentapeptide chains of the cell wall peptidoglycan during cell wall synthesis, resulting in slower growth of the resistant isolates. This phenomenon leads to a heteroresistant population, wherein cells exhibit different levels of resistance and some are phenotypically susceptible 114 . The above-mentioned susceptibility testing guidelines enable the slower growing MRSA subpopulation to reach detectable levels in a heteroresistant population. Rarely, MRSA may present with phenotypic sensitivity to cefoxitin (and oxacillin) and require an overnight exposure to low concentrations of cefoxitin to exhibit resistance 115 . In this case, the presence of inducible mecA should be considered. Methicillin resistance in S. aureus colonies and cultures can also be detected by means of an antigen–antibody-based latex agglutination test that detects PBP2a by using an anti-PBP2a antibody. Moreover, several automated instruments performing identification and antimicrobial susceptibility testing of staphylococci have shown high sensitivities and specificities for the MRSA strains tested (reviewed in Ref. 116 ).
For direct phenotypic detection of MRSA from positive blood cultures, there is renewed interest in refining bacteriophage-based assays. The KeyPath MRSA/MSSA blood culture test (MicroPhage Inc, Longmont, Colorado, USA) is a US FDA-approved, non-genotypic, rapid test for the identification of S. aureus and the detection of methicillin resistance directly from positive blood cultures. The assay detects the amplification of S. aureus -specific bacteriophages in the presence of methicillin with a turnaround time of 5 hours. Multicentre evaluation of this assay on 1,116 blood cultures showed 91.8% sensitivity, 98.3% specificity, 96.3% positive predictive value and 96.1% negative predictive value, with a median turnaround time of 16.9 hours versus 46.9 hours calculated for conventional tests for the identification of S. aureus and differentiating between MRSA and MSSA in positive blood cultures 117 .
Non-phenotypic methods. One of the most promising non-genotypic techniques for direct identification of pathogens from positive blood cultures is matrix-assisted laser desorption-ionization time-of-flight mass spectrometry (MALDI-TOF MS) 118 . Identification is based on the comparison of the protein profile obtained by mass spectrometry from a bacterial or fungal sample with a database of profiles obtained from several characterized microorganisms. However, as the performance of MALDI-TOF MS largely depends on a microorganism's purity and quantity, bacterial enrichment and purification procedures are required from positive blood cultures, which contain high concentrations of interfering non-microbial material 118 . A retrospective study of 227 cases of S. aureus bacteraemia comparing turnaround time and therapy adjustment before and after the introduction of MALDI-TOF MS plus real-time PCR to detect mecA showed a decrease in turnaround time of MRSA identification by nearly 50% compared with S. aureus identification and β-lactam susceptibility testing by conventional methods. Although the length of hospitalization and rates of adequate empirical antibacterial therapy were similar in the two groups, optimization of therapy occurred more frequently in the group assessed by MALDI-TOF MS 119 .
Current DNA-based methods for direct MRSA detection from clinical samples are multiplex real-time PCR assays to detect S. aureus and the presence of mecA 120 and are well-validated assays 121 . Results are obtained in approximately 1.5 hours. The FilmArray (Idaho Technology, Salt Lake City, Utah, USA) is a multiplex PCR-based system designed to detect 25 microorganisms (90–95% of the pathogens involved in blood cultures) along with mecA , as well as the presence of genes encoding resistance to vancomycin ( vanA and vanB ) and carbapenems ( bla KPC ) 122 . This assay has higher sensitivity than MALDI-TOF MS in identifying microorganisms from blood culture bottles before positivity, with an average turnaround time of 2.5 hours 123 .
The application of WGS to bacterial pathogens heralded the single most important advance in diagnostic microbiology and surveillance since in vitro culture. However, direct applications of WGS in diagnostic microbiology remain limited, primarily because of the technological constraints in obtaining results within a time frame that can influence patient care and the need for standardized protocols and automated data interpretation. The introduction of the third generation of sequencers (such as the Oxford Nanopore MinION by Pacific BioSciences and Oxford Nanopore, Oxford, UK) has resulted in longer reads (obtained sequence lengths) that can span repeat regions in the bacterial sequence and enable complete bacterial genome assembly, as well as an increased portability of the machinery and a potential reduction in error rates. An important benefit afforded by the Oxford Nanopore MinION sequencer is that sequencing data can be analysed in real time and could lead to strain identification within 30 min and an antibiotic-resistance profile prediction within 10 hours after the start of a run 124 , making this assay potentially useful for clinical diagnostics. The utility of WGS has been well demonstrated for studying antibiotic resistance and the population biology of MRSA 125 and has also led to many useful insights regarding transmission of MRSA during hospital outbreaks 126 and in community settings 127 .
Screening methods
Screening measures and their effectiveness are discussed in the Prevention section below. Since the introduction of the first MRSA chromogenic medium (that is, a medium containing synthetic chromogenic enzyme substrates; in the presence of the specific target enzyme, the chromogenic substrate is processed and results in a corresponding bacterial colony of a specific colour, thereby enabling pathogen recognition) 128 , these media have undergone rapid improvements in terms of sensitivity of the chromogen and the antibiotics used 120 , 129 . They have become the primary rapid diagnostic assays utilized for active surveillance for MRSA colonization as well as for patient diagnostics since they were introduced in the 2000s 129 . In 2005, an external quality assessment in 23 European countries and Israel found that 88% of the participating laboratories utilized a chromogenic medium alone to screen for MRSA 130 . The combination of chromogenic media with MALDI-TOF MS, which enables the species identification of multiple colonies in <1 hour, has further improved the specificity and turnaround time 131 . Development of automated colony scoring that could further increase specificity and reduce turnaround time is also being attempted 132 .
Application of real-time PCR-based assays for MRSA screening from nasal swabs can decrease turnaround time to 1–2 hours, whereas the results of chromogenic media-based tests can take a minimum of 14–18 hours without confirmatory testing and, therefore, might not always be useful to guide clinical decisions. An observational cohort study demonstrated a significant reduction in MRSA transmission upon screening with a same-day commercial real-time PCR assay compared with screening with conventional culture (swabs incubated overnight in 7% NaCl and subcultured on mannitol salt agar with 2 milligrams per litre oxacillin for 48 hours): MRSA transmission was 4.9 new acquisitions per 1,000 patient bed days with real-time PCR compared with 13.9 new acquisitions per 1,000 patient bed days with culture 133 . (A patient bed day represents a unit of time during which a patient occupies a bed and stays overnight in a health-care facility; thus, 50 patients in a hospital over a period of 1 day would represent 50 patient bed days.) However, a major study in 13 ICUs in eight European countries did not find any positive effect of screening using PCR-based tests versus chromogenic media in the acquisition and transmission rates of multidrug-resistant bacteria (including MRSA, vancomycin-resistant enterococci and highly-resistant Enterobacteriaceae) 134 . Similarly, a UK-based study assessing screening by real-time PCR-based tests versus slower laboratory-based methods (MRSA-selective broth and chromogenic medium) reported a significant reduction in turnaround times (from 40.4 to 3.7 hours) but again no effect on MRSA acquisition rates 135 , thereby rendering the utility of the more-expensive albeit faster PCR-based screening questionable.
MRSA control interventions have been widely implemented across health-care facilities. These interventions aim to limit the emergence of MRSA by facilitating judicious use of antimicrobial agents (including introducing restrictions on their prescription), control the reservoir of patients who are carriers, prevent MRSA transmission between patients and prevent the development of infection in carriers. Several measures are usually required to successfully prevent transmission and infection with MRSA 136 . Decolonization, an important control intervention for which there is growing evidence, is discussed in the Management section.
Hand hygiene. By contact with patients with MRSA colonization or handling MRSA-contaminated equipment, health-care workers can acquire MRSA on their hands, and by this means, MRSA can be transmitted between patients 137 . Hand hygiene, with alcohol-based hand rub or soap and water, aims to reduce MRSA spread via this route. Indeed, the WHO has identified hand hygiene as an important factor in providing safe patient care and has issued detailed instructions regarding appropriate hand hygiene practices among health-care workers 137 , 138 . The effectiveness of improving compliance with hand hygiene among health-care workers in MRSA control has been demonstrated at local as well as national levels 139 , 140 . For example, the roll out of a national hand hygiene programme in England and Wales from late 2004 was associated with a fall in the incidence of MRSA bacteraemia from 1.88 to 0.91 per 10,000 patient bed days 140 . Although the hand hygiene campaign was implemented with other national infection control initiatives, the higher procurement of alcohol hand rub during the campaign was independently associated with reduction in the incidence of MRSA bacteraemia after adjustment for all other interventions 140 .
Active surveillance. Most patients with MRSA colonization are asymptomatic, and, therefore, relying on culture of clinical samples (which are collected only when an individual develops symptoms or signs of infection) alone to identify carriers of MRSA may fail to identify up to 85% of individuals with MRSA colonization 141 . Through screening methods, active surveillance programmes can identify this large asymptomatic reservoir of carriers and direct interventions (such as topical decolonization) to reduce transmission or infection risk. MRSA screening may be universal (applied to all patients) or targeted (limited to patients at increased risk of MRSA carriage). Universal MRSA screening has been one of the most controversial areas in infection control since the 2000s, with some studies showing that it is effective in reducing MRSA-associated disease 142 , whereas other studies found it ineffective 143 , 144 . Importantly, recent data also show that universal screening is unlikely to be cost-effective, particularly in settings with low or decreasing MRSA prevalence 145 . On the basis of this accumulating evidence, many health-care facilities have now abandoned universal MRSA screening. We suggest, however, that changes in practices should be based on careful consideration of local MRSA epidemiology and the vulnerability of the patient population.
Randomized trials in ICUs have questioned the utility of routine MRSA screening in this high-risk setting 146 , 147 . However, a long turnaround time for screening results (a mean of 5.2 days with a culture-based method using a pre-enrichment step at a central laboratory) 146 or established good hand hygiene practices coupled with universal chlorhexidine bathing 147 might have contributed to the observed lack of effect of screening. It is argued that ‘horizontal’ strategies (that is, strategies aimed at preventing all health-care-associated infections, including MRSA, such as hand hygiene and universal bathing with antiseptics) are a better use of limited resources.
Contact precautions and isolation. In many facilities, health-care workers use contact precautions (use of disposable gowns and gloves) when caring for patients with MRSA colonization to reduce MRSA transmission associated with contamination of hands and clothing. Although the evidence for this intervention has previously been of low quality, there is now more robust data suggesting that this practice is associated with reduction in MRSA acquisition 148 . It is also widely recommended that patients with MRSA colonization are isolated in single rooms. However, in a prospective study in an ICU setting where MRSA was endemic and hand hygiene compliance was low, single-room isolation was not effective in reducing MRSA transmission 149 . Experts have called for a review of this practice and for guidelines to highlight the uncertainties regarding its value 150 .
The approach to the management of MRSA varies in different geographical regions depending on local MRSA prevalence and availability of antimicrobials, particularly the newer agents.
Decolonization of carriers
MRSA colonization is associated with an increased risk of infection and contributes to transmission. Both MRSA colonization on admission as well as acquisition during hospitalization are associated with an approximately tenfold increased risk of subsequent infection 107 . Thus, decolonization can contribute to MRSA control by reducing transmission and infection risk. Most decolonization strategies use topical agents applied to the nostrils, the principal site of colonization 47 . Mupirocin (pseudomonic acid A, which inhibits bacterial isoleucyl tRNA synthetase, preventing protein synthesis) is the principal agent and is often combined with chlorhexidine bathing 151 . Although mupirocin is the cornerstone for eradication of S. aureus , resistance is increasing, with some studies reporting resistance rates of up to 80% in MRSA 152 . Alternative agents are being studied, but to date, experience with these agents is limited 153 . Thus, it is recommended that mupirocin is used judiciously and that the emergence of resistance is monitored 152 . Ongoing research into the development and evaluation of new agents that can be effectively used for decolonization is also needed.
Short-term decolonization. Decolonization is most commonly used as a protective strategy during relatively short periods of increased risk of infection, for example, during the peri-operative period or ICU stays. Topical mupirocin to the nares and chlorhexidine body washing before surgery for known S. aureus carriers reduced the risk of post-surgical S. aureus infection by ∼ 50% in a placebo-controlled study 154 . A subsequent cost-effectiveness analysis using these data showed that the mean cost saved per treated carrier was €1,911 (Ref. 155 ). However, this study was performed in the Netherlands, a country with low MRSA prevalence, and the effects of this short-term decolonization strategy might be different in settings with high MRSA prevalence.
The results of short-term decolonization interventions in the ICU setting have been variable. A large cluster-randomized trial compared three strategies 156 : screening and isolation of MRSA carriers (no decolonization); a combination of screening, isolation and decolonization (with mupirocin and chlorhexidine bathing) of MRSA carriers (targeted decolonization); and decolonization of all patients (universal decolonization). No significant differences in MRSA colonization and infection rates were found with the three strategies. However, bloodstream infections from any pathogen were significantly lower in the universal decolonization group. This may have been the result of universal chlorhexidine bathing rather than mupirocin. The authors concluded that universal decolonization was the best approach, as it reduced infections overall without the need for screening. However, as widespread use of topical antibiotics might lead to an increase in drug resistance, their use should be coupled with monitoring for resistance 152 .
Permanent decolonization. In some situations, permanent MRSA eradication is pursued. Permanent decolonization is a component of the ‘search and destroy strategy’ in countries with a low MRSA prevalence 157 . For example, the Dutch protocol distinguishes between uncomplicated and complicated carriers on the basis of MRSA strain and host characteristics, as well as colonization site, as treatment failure is three times more likely in individuals with throat colonization than in those without throat colonization 158 . Of 613 MRSA carriers, 80% were ultimately successfully decolonized, with a median time to decolonization of 10 days; of note, adherence to the protocol was crucial for success. Many other clinical trials evaluating permanent decolonization strategies have been conducted under real-life conditions, with rather disappointing results because of a high rate of endogenous recolonization 151 .
Treatment of symptomatic infection
Empirical treatment and SSTIs. We recommend that an antibiotic effective against MRSA should be considered for empirical treatment of infection for patients with several risk factors for HA-MRSA infection or those with presumed severe staphylococcal infections in settings where MRSA prevalence is >20%, although precise thresholds have not been established. The choice, route of administration and duration of antibiotic therapy are determined by the site and severity of infection. Treatment should then be adjusted on the basis of subsequent results of cultures and susceptibility testing.
Intravenous vancomycin, daptomycin or linezolid can be used for severe SSTIs. Oral therapy as a rule should be avoided in the initial treatment of severe infections. Clindamycin, trimethoprim–sulfamethoxazole and doxycycline are alternative choices for the treatment of mild to moderate SSTIs, depending upon the antibiotic susceptibility testing. For uncomplicated skin abscesses, the use of clindamycin or trimethoprim–sulfamethoxazole in conjunction with incision and drainage has been shown to improve clinical cure rates in the emergency department and other outpatient settings 159 , 160 .
Systemic and severe infections. The current recommendations for clinical management of severe MRSA infections include intravenous vancomycin or daptomycin for bacteraemia and intravenous vancomycin or linezolid for hospital-acquired pneumonia 161 . For severe infections, oral linezolid should not be used for initial therapy. However, when the patient has become stable and can tolerate the oral route, a switch to oral linezolid is recommended. In the setting of infection related to the presence of a medical device (such as central venous catheters), successful treatment usually requires removal of the device when possible 161 .
Glycopeptides (such as vancomycin and teicoplanin) have been the mainstay of intravenous treatment for MRSA infections. Vancomycin remains the cornerstone of empirical treatment for systemic infections potentially caused by MRSA, first because of its safety profile and second owing to lack of other fully approved alternatives 161 , 162 . Teicoplanin is also commonly used in Europe and has been found to be non-inferior to vancomycin in terms of all-cause mortality, with an improved safety profile, although few patients with serious infections were studied 163 ( Table 3 ). Of note, glycopeptides have slower bactericidal activity than β-lactam agents, and penetration into tissues is poor.
Recommendations have been made to increase vancomycin administration to achieve an appropriate ‘trough’ concentration (lowest concentration reached by the drug before the next dose is administered) and, in this way, to maximize the chances of microbiological and clinical cure (eradication of the organism as demonstrated by negative cultures and resolution of signs and symptoms of infection, respectively) 161 , 164 . Also, optimization of vancomycin therapy on the basis of pharmacokinetic and pharmacodynamic targets is becoming increasingly relevant, particularly as reports of the incidence of MRSA clinical isolates with minimum inhibitory concentrations (MICs) >1 microgram per millilitre (which is just below the breakpoint) are increasing in several settings. However, higher trough concentrations are associated with an increased risk of nephrotoxicity 165 and no clear improvement in outcome. Finally, vancomycin is administered in a continuous infusion instead of intermittent injections in some European countries 166 . However, there are insufficient data to make recommendations regarding this protocol 166 . In the presence of infections caused by MRSA strains with an MIC higher than the current breakpoint (>2 micrograms per millilitre), vancomycin is not effective, and an alternative agent should be administered 167 . Switching to daptomycin therapy, on the basis of the daptomycin MIC, should be done as early as possible once an elevated vancomycin MIC is confirmed. Alternative anti-MRSA antibiotics are increasingly being used, but it is important to note that they can have adverse effects, particularly linezolid 168 ( Table 3 ). Of note, although reports of vancomycin failure have emerged for vancomycin intermediate-resistant S. aureus (VISA) and/or heterogeneous VISA (hVISA) infections, no data demonstrate superior outcomes with alternative antimicrobials agents ( Box 1 ).
Combination therapy. The duration of bacteraemia in patients with MRSA is twice as long as that in patients with MSSA infection 169 . The increased duration of bacteraemia is associated with complications (such as attributable mortality, complicated infection, embolic stroke or recurrent S. aureus infection) 170 . Combination therapy to treat S. aureus (including MRSA) bacteraemia has been used in an attempt to increase bacterial killing, particularly for endocarditis therapy 171 . However, evidence that combination therapy improves outcomes is lacking 171 . Several studies have demonstrated in vitro synergy between vancomycin and gentamicin against many MRSA isolates 172 , 173 . However, this combination seemed to be numerically inferior to daptomycin alone in the treatment of MRSA bacteraemia and endocarditis in a randomized trial 174 . Thus, because even low dose gentamicin (1 milligram per kilogram every 8 hours) for a short duration has been associated with substantial nephrotoxicity 175 and because the clinical effectiveness of vancomycin plus gentamicin is not confirmed, combination therapy with aminoglycosides is difficult to justify 176 .
Vancomycin and rifampicin combinations have also been studied, particularly in the context of biofilm infections 177 . However, the addition of rifampicin to vancomycin is not recommended for MRSA bacteraemia or native valve endocarditis 161 . In addition, a randomized controlled trial evaluating adjunctive rifampicin in S. aureus (including MRSA) bacteraemia found no overall benefit 178 .
The combination of vancomycin and β-lactam antibiotics has shown synergistic bacterial killing in vitro 179 . However, sufficient clinical evidence in favour of this combination is lacking. In the CAMERA-1 trial (comparing vancomycin versus vancomycin plus flucloxacillin), the mean time to resolution of bacteraemia (primary outcome) in the combination group was 1.94 days, compared with 3.00 days in the vancomycin alone group. According to a negative binomial model, the mean time to resolution of bacteraemia in the combination group was 65% (95% CI 41–102%; P = 0.06) of that in the group that received intravenous vancomycin alone (that is, it was 35% lower), and there was no difference between the two groups in relation to the secondary end points 179 .
Ceftaroline plus daptomycin could be another option for refractory staphylococcal bacteraemia. Ceftaroline offers dual benefit via synergy with daptomycin and sensitization to cathelicidin antimicrobial peptide-derived LL-37, a peptide of the host innate immune response; sensitization to cathelicidin could attenuate the virulence of the pathogen 180 (see New drugs and current pipeline). Other combinations (for example, daptomycin and rifampicin) might be promising options in biofilm-related infections 181 .
Other considerations. In contrast to many other bacterial infections, S. aureus (including MRSA) infections often require a lengthy course of treatment because of the risk of late-onset complications such as abscesses, osteoarticular infection and other secondary foci caused by haematogenous or direct seeding. In cases of documented bacteraemia, the recommended minimum duration of treatment is 14 days 161 , as short-course therapy is currently not considered to be safe and effective.
Options for salvage therapy, based on low-quality data, include linezolid, trimethoprim–sulfamethoxazole, ceftaroline, quinupristin–dalfopristin and telavancin. Tigecycline should be avoided, as it is bacteriostatic against MRSA and has a large volume of distribution with high concentrations in tissues but low concentrations in serum 161 . No data in MRSA bacteraemia are yet available for other recently approved agents (for example, ceftobiprole, dalbavancin, oritavancin or tedizolid).
New drugs and current pipeline
Several new agents have been approved for the treatment of SSTIs and in some cases for pneumonia ( Fig. 5 ; Table 4 ). However, the efficacy and safety of these antibiotics for invasive infections, for which there is a real need, have largely not been demonstrated. The new treatment options have some advantages over older agents and will probably play a part in the therapy of severe MRSA infections in the near future.
Ceftaroline and ceftobiprole are the first β-lactams with anti-MRSA activity. Use of the combination of daptomycin with an anti-staphylococcal β-lactam for refractory MRSA infections has been increasing. Multiple case reports have now documented success of the daptomycin–ceftaroline combination for MRSA bacteraemia and endocarditis 182 . Ceftobiprole for endocarditis has been studied in animal models only, with promising results 183 , with a single human case report of ceftobiprole plus daptomycin for MRSA endocarditis 184 . However, resistance to ceftaroline has been observed in MRSA strains 185 , 186 . Of particular concern are reports of resistance in clinical MRSA isolates from patients in geographical regions never exposed to the drug 187 .
Teicoplanin, introduced in Europe in 1988, was the first natural agent of the lipoglycopeptides class. Synthetic and semisynthetic derivatives of lipoglycopeptides have been produced, including telavancin in 2009 and dalbavancin and oritavancin in 2014 (Ref. 188 ). In 10 years of dalbavancin surveillance testing, only 0.35% of S. aureus isolates exceeded the FDA susceptibility breakpoint and, therefore, were dalbavancin nonsusceptible 189 . However, the long half-life of dalbavancin could lead to prolonged periods of low-level drug exposure at the end of therapy, potentially increasing the risk of resistance selection 190 .
Possible advantages of tedizolid over linezolid include once-daily dosing, better adverse effects profile and lower risk of development of spontaneous resistance and susceptibility to the ribosomal RNA large subunit methyltransferase Cfr mobile resistance mechanism. As experience increases, tedizolid may be an attractive alternative for long-term treatment of osteoarticular and central nervous system infections. Trials in pneumonia are underway.
Ceftaroline, ceftobiprole, telavancin, dalbavancin and delafloxacin all require renal dose adjustment (that is, the antibiotic dose requires modification in individuals with impaired renal function).
Quality of life
Clinical effect.
MRSA infections frequently complicate medical care and cause important treatment challenges. Most experts agree that β-lactam antibiotics are the optimal choice for treating invasive staphylococcal infections 171 . Thus, MRSA treatment relies on less efficacious (vancomycin) or more expensive (daptomycin or linezolid) therapeutic options. Owing to important prognostic cofactors such as potentially inadequate treatment, patient comorbidities and underlying illness, MRSA infections tend to have higher morbidity and mortality than MSSA infections 191 .
However, the true clinical effect of methicillin resistance in S. aureus infection has been overestimated owing to methodological shortcomings of studies 192 . More-recent studies using advanced analytical approaches accounting for potential confounders and competing events confirm a non-negligible effect, which is, however, less pronounced than previously hypothesized 193 , 194 . For instance, a large international retrospective cohort study in ten European hospitals with endemic MRSA reported that methicillin resistance in S. aureus bacteraemia was not significantly associated with increased probability of in-hospital mortality (adjusted HR 1.26; 95% CI 0.82–1.94) after adjusting for potential confounders and accounting for the timing of events 195 . In this study, both MSSA and MRSA bacteraemia led to prolonged length of hospital stay, with a nonsignificant difference of 2.5 days (95% CI –3.2 to 8.3) longer for patients with MRSA infection.
Among patients with bacteraemia caused by S. aureus , methicillin resistance is associated with adverse health outcomes. Several studies show that patients with invasive MRSA infection might have diminished probability of long-term survival, regardless of the adequacy of initial treatment 191 , 196 . Furthermore, patients with MRSA infection can experience post-infection sequelae (such as the requirement for amputation owing to the higher risk of treatment failure for MRSA infections of prosthetic joints) and harmful adverse effects related to MRSA treatment 197 , 198 . Family members might be overwhelmed with caring for patients with MRSA infection in the community 199 . By contrast, MRSA carriage may not cause major concern per se, as shown in a Dutch study in which health-related quality of life was not decreased in otherwise healthy pig farmers carrying LA-MRSA 200 .
Burden of disease
MRSA infections add to the global burden of antibiotic resistance. Several studies have shown that increased incidence of HA-MRSA infection occurs in addition to infections caused by MSSA, increasing the total burden of disease 201 . Furthermore, once endemic levels of MRSA are reached in a clinical setting, physicians are required to treat patients empirically for MRSA in cases of severe nosocomial infection 161 . This probabilistic approach of adding vancomycin or linezolid to empiric antibiotic coverage might be continued for years, even after MRSA incidence has substantially declined 202 . Finally, MRSA control requires substantial infrastructure and productivity costs of surveillance, screening and isolation of MRSA carriers 203 . However, these MRSA control expenditures (for example, universal, nation-wide MRSA screening) may no longer be justified once MRSA prevalence has declined below specific thresholds 145 .
The development of a vaccine could have an enormous effect on the incidence and outcome of MRSA infections. Indeed, S. aureus carriers have more frequent infections, but the infections are less severe than those developed by non-carriers 204 , indicating that long-term exposure to S. aureus antigens can lead to protective immunity. A vaccine could prevent infections at the onset and would ideally also impair S. aureus colonization, thereby strongly reducing the need for antibiotic treatment and extensive infection control measures 205 . Therapeutic monoclonal antibodies such as the passive vaccine against α-haemolysin by Medimmune (Gaithersburg, Maryland, USA) could provide new treatment opportunities, either alone or in combination with antibiotics. However, despite extensive research and development efforts, a protective vaccine against S. aureus will not become available in the next few years.
Two monovalent vaccine candidates have previously been tested but failed to induce protective immunity in late clinical development. The StaphVax vaccine (Nabi Biopharmaceuticals, Rockville, Maryland, USA), containing the capsular polysaccharide 5 (CP5) and CP8 antigens, and the V710 (Merck, Kenilworth, New Jersey, USA) vaccine, containing the iron-regulated surface determinant protein B (IsdB) antigen, have been protective in animal models but not in placebo-controlled phase III trials 206 , 207 . The reasons for failure remain unclear but may be related to the fact that several important S. aureus clones, including the major MRSA clone USA300, do not express any CPs, that the antigen preparations lacked adjuvants and that immune responses to the antigens used were not consistent enough. Moreover, there is a general concern that the extensive set of S. aureus immune evasion factors, such as immunoglobulin G (IgG)-binding protein A, could compromise the efficacy of antibodies, that opsonizing antibodies might not be sufficient to promote protection, whereas toxin-neutralizing antibodies might be equally or even more important, and that appropriate T cell-mediated immunity might be more crucial than previously thought 208 .
Advances from basic science provide cues for new and hopefully more-successful vaccination approaches. Immunoproteomics studies have helped to elucidate the most immunogenic and protective S. aureus antigens 209 , and the cell wall glycopolymer wall teichoic acid (WTA) has been identified as a dominant surface antigen 210 . Several new toxins, whose neutralization by antibodies might contribute to protection, have been identified 64 . Moreover, it has become clearer which T cell subsets are required for anti- S. aureus immunity 211 . Pharmaceutical companies continue to develop polyvalent anti- S. aureus vaccines based on surface proteins (ClfA) and polysaccharides (CP5 and CP8), secreted toxins (α-toxin, LukS-PV, ESAT-6 secretion system extracellular protein A (EsxA) and EsxB) and membrane-bound lipoproteins involved in nutrient uptake (manganese transport system membrane protein MntC and ferric hydroxamate receptor 2 (Fhud2)) 205 . An innovative WTA-targeting monoclonal antibody conjugated to a rifampicin-related antibiotic showed protection in preclinical infection models 212 . There is hope that some of the ongoing vaccine development efforts may lead to successful completion of clinical trials.
Research needs and priorities
MRSA will probably always coexist with humanity. Despite the current focus on multidrug-resistant Gram-negative bacteria and the decline of HA-MRSA infections in some regions, the biomedical research community would be well advised not to abandon its diverse activities in the field of MRSA research. As highlighted by a 2017 WHO report 213 , MRSA remains among the high-priority multidrug-resistant organisms that need renewed efforts for the research and development of new antibiotics and innovative preventive approaches. In addition to protective vaccines, bacteriophages or bacteriophage-derived lytic proteins could be used for new protective strategies, for instance, for nasal MRSA decolonization in an era of increasing mupirocin resistance 214 . Overall, there are still many knowledge gaps and important challenges to tackle ( Box 2 ), which require ongoing attention from researchers, policy makers and funders as well as those responsible for MRSA treatment and control.
MRSA has demonstrated its remarkable ability to evolve and disseminate widely in the 60 years since it was first recognized. Several factors, including a better understanding of the pathogenesis of infection, accurate and rapid diagnostics, ensuring the availability of effective treatment options and optimization of the prevention of transmission and infection, will ultimately facilitate control of this highly successful pathogen.
Box 2: Future research needs
Life cycle and survival fitness of successful methicillin-resistant Staphylococcus aureus (MRSA) clones: prediction modelling and simulation of future introduction of successful community-associated MRSA strains into the health-care environment
Virulence mechanisms: development of new inhibition strategies targeted to prevention and treatment of invasive MRSA infections
Interplay between the host, S. aureus and competing nasal microbiota: microbiome studies on the competition between S. aureus and other commensal microbiota
New decolonization regimens and approaches to decrease risk of MRSA infection: development and clinical evaluation of new drugs, vaccines and other preventive strategies
Novel treatment approaches: discovery of inhibitors of the S. aureus stringent stress response or other S. aureus factors that can sensitize MRSA to β-lactam antibiotics 103
Whole-genome sequencing (WGS): effectiveness studies on WGS usefulness for clinical diagnostics and rapid antibiotic susceptibility testing
Biomarkers: rapid molecular diagnostic tools and biomarkers for individual risk-profiling and treatment approaches
MRSA reservoirs in animals: determinants of MRSA host tropism and host jumps
Publisher's note
Springer Nature remains neutral with regard to jurisdictional claims in published maps and institutional affiliations.
Reviewer information
Nature Reviews Disease Primers would like to thank L. Cui, P. Wilson and other anonymous reviewer(s) for their contribution to the peer review of this work.
Related links
CDDEP Resistance Map
ClinicalTrials.gov
European Medicines Agency
International Working Group on the Staphylococcal Cassette Chromosome elements
List of Prokaryotic names with Standing in Nomenclature
Surveillance Atlas of Infectious Diseases
US Food and Drug Administration
Wertheim, H. F. et al. The role of nasal carriage in Staphylococcus aureus infections. Lancet Infect. Dis. 5 , 751–762 (2005).
PubMed Google Scholar
Becker, K. et al. Staphylococcus aureus from the German general population is highly diverse. Int. J. Med. Microbiol. 307 , 21–27 (2017).
CAS PubMed Google Scholar
Kuehnert, M. J. et al. Prevalence of Staphylococcus aureus nasal colonization in the United States, 2001–2002. J. Infect. Dis. 193 , 172–179 (2006).
Lowy, F. D. Staphylococcus aureus infections. N. Engi J. Med. 339 , 520–532 (1998).
CAS Google Scholar
Jevons, M. “Celbenin”-resistant staphylococci. BMJ 1 , 124–125 (1961).
PubMed Central Google Scholar
Dzintars, K. in Kucers’ The Use of Antibiotics (eds Grayson, M. et al.) 136–142 (CRC Press, 2018).
Google Scholar
Chambers, H. F. & Deleo, F. R. Waves of resistance: Staphylococcus aureus in the antibiotic era. Nat. Rev. Microbiol. 7 , 629–641 (2009). This is an in-depth review of the clinical and molecular epidemiology of S. aureus , including MRSA, with an emphasis on CA-MRSA.
CAS PubMed PubMed Central Google Scholar
Faoagali, J. L., Thong, M. L. & Grant, D. Ten years’ experience with methicillin-resistant Staphylococcus aureus in a large Australian hospital. J. Hosp. Infect. 20 , 113–119 (1992).
Fridkin, S. K. et al. Methicillin-resistant Staphylococcus aureus disease in three communities. N. Engl. J. Med. 352 , 1436–1444 (2005).
Voss, A., Loeffen, F., Bakker, J., Klaassen, C. & Wulf, M. Methicillin-resistant Staphylococcus aureus in pig farming. Emerg. Infect. Dis. 11 , 1965–1966 (2005).
PubMed PubMed Central Google Scholar
Tenover, F. C. et al. Interpreting chromosomal DNA restriction patterns produced by pulsed-field gel electrophoresis: criteria for bacterial strain typing. J. Clin. Microbiol. 33 , 2233–2239 (1995).
Ito, T., Katayama, Y. & Hiramatsu, K. Cloning and nucleotide sequence determination of the entire mec DNA of pre-methicillin-resistant Staphylococcus aureus N315. Antimicrob. Agents Chemother 43 , 1449–1458 (1999).
Harkins, C. P. et al. Methicillin-resistant Staphylococcus aureus emerged long before the introduction of methicillin into clinical practice. Genome Biol. 18 , 130 (2017).
Diekema, D. J. et al. Survey of infections due to Staphylococcus species: frequency of occurrence and antimicrobial susceptibility of isolates collected in the United States, Canada, Latin America, Europe, and the Western Pacific region for the SENTRY Antimicrobial Surveillance Program, 1997–1999. Clin. Infect. Dis. 32 (Suppl. 2), S114–S132 (2001).
Enright, M. C., Day, N. P., Davies, C. E., Peacock, S. J. & Spratt, B. G. Multilocus sequence typing for characterization of methicillin-resistant and methicillin-susceptible clones of Staphylococcus aureus. J. Clin. Microbiol. 38 , 1008–1015 (2000).
Monecke, S. et al. A field guide to pandemic, epidemic and sporadic clones of methicillin-resistant Staphylococcus aureus. PLoS ONE 6 , e17936 (2011).
[No authors listed.] European Antimicrobial Resistance Surveillance Network (EARS-Net). European Centre for Disease Prevention and Control http://ecdc.europa.eu/en/activities/surveillance/EARS-Net/Pages/index.aspx (2018).
Kock, R. et al. Methicillin-resistant Staphylococcus aureus (MRSA): burden of disease and control challenges in Europe. Eurosurveillance 15 , 19688 (2010).
Nielsen, K. L. et al. Fitness cost: a bacteriological explanation for the demise of the first international methicillin-resistant Staphylococcus aureus epidemic. J. Antimicrob. Chemother. 67 , 1325–1332 (2012).
Rolain, J. M., Abat, C., Brouqui, P. & Raoult, D. Worldwide decrease in methicillin-resistant Staphylococcus aureus: do we understand something? Clin. Microbiol. Infect. 21 , 515–517 (2015).
Garcia- Alvarez, L., Dawson, S., Cookson, B. & Hawkey, P. Working across the veterinary and human health sectors. J. Antimicrob. Chemother. 67 (Suppl. 1), Í37–Í49 (2012).
van Alen, S. et al. In the centre of an epidemic: fifteen years of LA-MRSA CC398 at the University Hospital Munster. Vet. Microbiol. 200 , 19–24 (2017).
Lozano, C. et al. High prevalence of spa types associated with the clonal lineage CC398 among tetracycline-resistant methicillin-resistant Staphylococcus aureus strains in a Spanish hospital. J. Antimicrob. Chemother. 67 , 330–334 (2012).
van Cleef, B. A. et al. Livestock-associated methicillin-resistant Staphylococcus aureus in humans, Europe. Emerg. Infect. Dis. 17 , 502–505 (2011).
Larsen, J. et al. Meticillin-resistant Staphylococcus aureus CC398 is an increasing cause of disease in people with no livestock contact in Denmark, 1999 to 2011. Eurosurveillance 20 , 30021 (2015).
Hetem, D. J., Bootsma, M. C., Troelstra, A. & Bonten, M. J. Transmissibility of livestock-associated methicillin-resistant Staphylococcus aureus. Emerg. Infect. Dis. 19 , 1797–1802 (2013).
Styers, D., Sheehan, D. J., Hogan, P. & Sahm, D. F. Laboratory-based surveillance of current antimicrobial resistance patterns and trends among Staphylococcus aureus: 2005 status in the United States. Ann. Clin. Microbiol. Antimicrob. 5 , 2 (2006).
Talan, D. A. et al. Comparison of Staphylococcus aureus from skin and soft-tissue infections in US emergency department patients, 2004 and 2008. Clin. Infect. Dis. 53 , 144–149 (2011).
Moran, G. J. et al. Methicillin-resistant S. aureus infections among patients in the emergency department. N. Engl. J. Med. 355 , 666–674 (2006).
Seybold, U. et al. Emergence of community-associated methicillin-resistant Staphylococcus aureus USA300 genotype as a major cause of health care-associated blood stream infections. Clin. Infect. Dis. 42 , 647–656 (2006).
Popovich, K. J., Weinstein, R. A. & Hota, B. Are community-associated methicillin-resistant Staphylococcus aureus (MRSA) strains replacing traditional nosocomial MRSA strains? Clin. Infect. Dis. 46 , 787–794 (2008).
Planet, P. J. et al. Parallel epidemics of community-associated methicillin-resistant Staphylococcus aureus USA300 infection in North and South America. J. Infect. Dis. 212 , 1874–1882 (2015).
Von Dach, E. et al. Comparative genomics of community-associated methicillin-resistant Staphylococcus aureus shows the emergence of clone ST8-USA300 in Geneva, Switzerland. J. Infect. Dis. 213 , 1370–1379 (2016).
Dantes, R. et al. National burden of invasive methicillin-resistant Staphylococcus aureus infections, United States, 2011. JAMA Intern. Med. 173 , 1970–1978 (2013).
Jain, R. et al. Veterans Affairs initiative to prevent methicillin-resistant Staphylococcus aureus infections. N. Engl. J. Med. 364 , 1419–1430 (2011).
Lee, G. M. et al. Effect of nonpayment for preventable infections in U. S. hospitals. N. Engl. J. Med. 367 , 1428–1437 (2012).
Chen, C. J. & Huang, Y. C. New epidemiology of Staphylococcus aureus infection in Asia. Clin. Microbiol. Infect. 20 , 605–623 (2014).
Mendes, R. E. et al. Regional resistance surveillance program results for 12 Asia-Pacific nations (2011). Antimicrob. Agents Chemother. 57 , 5721–5726 (2013).
Lai, C. C., Lin, S. H., Sheng, W. H. & Hsueh, P. R. Decrease in the incidence of meticillin-resistant Staphylococcus aureus nosocomial bloodstream infections in Taiwan. Int. J. Antimicrob. Agents 41 , 591–592 (2013).
[No authors listed.] Australian Group on Antimicrobial Resistance (AGAR). AGAR Group http://www.agargroup.org.au/ (2018).
Mitchell, B. G., Collignon, P. J., McCann, R., Wilkinson, I. J. & Wells, A. A major reduction in hospital-onset Staphylococcus aureus bacteremia in Australia-12 years of progress: an observational study. Clin. Infect. Dis. 59 , 969–975 (2014).
Williamson, D. A., Coombs, G. W. & Nimmo, G. R. Staphylococcus aureus ‘Down Under’: contemporary epidemiology of S. aureus in Australia, New Zealand, and the South West Pacific. Clin. Microbiol. Infect. 20 , 597–604 (2014).
Falagas, M. E., Karageorgopoulos, D. E., Leptidis, J. & Korbila, I. P. MRSA in Africa: filling the global map of antimicrobial resistance. PLoS ONE 8 , e68024 (2013).
Brink, A., Moolman, J., da Silva, M. C., Botha, M. & National Antibiotic Surveillance Forum. Antimicrobial susceptibility profile of selected bacteraemic pathogens from private institutions in South Africa. S. Afr. Med. J. 97 , 273–279 (2007).
Jansen van Rensburg, M. J., Whitelaw, A. C. & Elisha, B. G. Genetic basis of rifampicin resistance in methicillin-resistant Staphylococcus aureus suggests clonal expansion in hospitals in Cape Town, South Africa. BMC Microbiol. 12 , 46 (2012).
Zervou, F. N., Zacharioudakis, I. M., Ziakas, P. D., Rich, J. D. & Mylonakis, E. Prevalence of and risk factors for methicillin-resistant Staphylococcus aureus colonization in HIV infection: a meta-analysis. Clin. Infect. Dis. 59 , 1302–1311 (2014).
Kluytmans, J., van Belkum, A. & Verbrugh, H. Nasal carriage of Staphylococcus aureus: epidemiology, underlying mechanisms, and associated risks. Clin. Microbiol. Rev. 10 , 505–520 (1997). This is a comprehensive review of staphylococcal nasal colonization including its clinical relevance.
Sim, B. L., McBryde, E., Street, A. C. & Marshall, C. Multiple site surveillance cultures as a predictor of methicillin-resistant Staphylococcus aureus infections. Infect. Control Hosp. Epidemiol. 34 , 818–824 (2013).
Eriksen, N. H., Espersen, F., Rosdahl, V. T. & Jensen, K. Carriage of Staphylococcus aureus among 104 healthy persons during a 19-month period. Epidemiol. Infect. 115 , 51–60 (1995).
Hoffler, U., Bulanda, M., Heczko, P. B. & Pulverer, G. A comparison of staphylococcal nasal carrier rates in Germany and Poland. Med. Microbiol. Immunol. 164 , 285–290 (1978).
Emonts, M. et al. Host polymorphisms in interleukin 4, complement factor H, and C-reactive protein associated with nasal carriage of Staphylococcus aureus and occurrence of boils. J. Infect. Dis. 197 , 1244–1253 (2008).
Lucet, J. C. et al. Carriage of methicillin-resistant Staphylococcus aureus in home care settings: prevalence, duration, and transmission to household members. Arch. Intern. Med. 169 , 1372–1378 (2009).
Burian, M. et al. Temporal expression of adhesion factors and activity of global regulators during establishment of Staphylococcus aureus nasal colonization. J. Infect. Dis. 201 , 1414–1421 (2010).
Weidenmaier, C. et al. Differential roles of sortase-anchored surface proteins and wall teichoic acid in Staphylococcus aureus nasal colonization. Int. J. Med. Microbiol. 298 , 505–513 (2008).
Wertheim, H. F. et al. Key role for clumping factor B in Staphylococcus aureus nasal colonization of humans. PLoS Med. 5 , e17 (2008).
O’Brien, L. M., Walsh, E. J., Massey, R. C., Peacock, S. J. & Foster, T. J. Staphylococcus aureus clumping factor B (ClfB) promotes adherence to human type I cytokeratin 10: implications for nasal colonization. Cell. Microbiol. 4 , 759–770 (2002).
Liu, C. M. et al. Staphylococcus aureus and the ecology of the nasal microbiome. Sci. Adv. 1 , e1400216 (2015).
Lemon, K. P. et al. Comparative analyses of the bacterial microbiota of the human nostril and oropharynx. mBio 1 , e00129–10 (2010).
Krismer, B. et al. Nutrient limitation governs Staphylococcus aureus metabolism and niche adaptation in the human nose. PLoS Pathog. 10 , e1003862 (2014).
Zipperer, A. et al. Human commensals producing a novel antibiotic impair pathogen colonization. Nature 535 , 511–516 (2016).
Kaspar, U. et al. The culturome of the human nose habitats reveals individual bacterial fingerprint patterns. Environ. Microbiol. 18 , 2130–2142 (2016).
Krismer, B., Weidenmaier, C., Zipperer, A. & Peschel, A. The commensal lifestyle of Staphylococcus aureus and its interactions with the nasal microbiota. Nat. Rev. Microbiol. 15 , 675–687 (2017). This review provides a detailed discussion of the complex interplay between S. aureus and other organisms that form part of the nasal microbiota.
Boyle-Vavra, S. et al. USA300 and USA500 clonal lineages of Staphylococcus aureus do not produce a capsular polysaccharide due to conserved mutations in the cap5 locus. mBio 6 , e02585–14 (2015).
Spaan, A. N., van Strijp, J. A. G. & Torres, V. J. Leukocidins: staphylococcal bi-component pore-forming toxins find their receptors. Nat. Rev. Microbiol. 15 , 435–447 (2017). This is a comprehensive overview of the various pore-forming protein toxins and their cognate host receptors.
Laabei, M. et al. Predicting the virulence of MRSA from its genome sequence. Genome Res. 24 , 839–849 (2014).
Thammavongsa, V., Kim, H. K., Missiakas, D. & Schneewind, O. Staphylococcal manipulation of host immune responses. Nat. Rev. Microbiol. 13 , 529–543 (2015).
Recker, M. et al. Clonal differences in Staphylococcus aureus bacteraemia-associated mortality. Nat. Microbiol. 2 , 1381–1388 (2017). This paper reveals how different S. aureus lineages have adopted different strategies to overcome host responses and cause severe pathology.
Kluytmans, J. A. et al. Nasal carriage of Staphylococcus aureus as a major risk factor for wound infections after cardiac surgery. J. Infect. Dis. 171 , 216–219 (1995).
von Eiff, C., Becker, K., Machka, K., Stammer, H. & Peters, G. Nasal carriage as a source of Staphylococcus aureus bacteremia. N. Engl. J. Med. 344 , 11–16 (2001).
Foster, T. J., Geoghegan, J. A., Ganesh, V. K. & Hook, M. Adhesion, invasion and evasion: the many functions of the surface proteins of Staphylococcus aureus. Nat. Rev. Microbiol. 12 , 49–62 (2014).
Vanhommerig, E. et al. Comparison of biofilm formation between major clonal lineages of methicillin resistant Staphylococcus aureus. PLoS ONE 9 , e104561 (2014).
Spaan, A. N., Surewaard, B. G., Nijland, R. & van Strijp, J. A. Neutrophils versus Staphylococcus aureus: a biological tug of war. Annu. Rev. Microbiol. 67 , 629–650 (2013).
Hanzelmann, D. et al. Toll-like receptor 2 activation depends on lipopeptide shedding by bacterial surfactants. Nat. Commun. 7 , 12304 (2016).
Cheng, A. G., DeDent, A. C., Schneewind, O. & Missiakas, D. A play in four acts: Staphylococcus aureus abscess formation. Trends Microbiol. 19 , 225–232 (2011).
Laarman, A., Milder, F., van Strijp, J. & Rooijakkers, S. Complement inhibition by gram-positive pathogens: molecular mechanisms and therapeutic implications. J. Mol. Med. 88 , 115–120 (2010).
Stapels, D. A. et al. Staphylococcus aureus secretes a unique class of neutrophil serine protease inhibitors. Proc. Natl Acad. Sci. USA 111 , 13187–13192 (2014).
Peschel, A. & Sahl, H. G. The co-evolution of host cationic antimicrobial peptides and microbial resistance. Nat. Rev. Microbiol. 4 , 529–536 (2006).
Loffler, B. et al. Staphylococcus aureus panton–valentine leukocidin is a very potent cytotoxic factor for human neutrophils. PLoS Pathog. 6 , e1000715 (2010).
Spaulding, A. R. et al. Staphylococcal and streptococcal superantigen exotoxins. Clin. Microbiol. Rev. 26 , 422–447 (2013).
Chavakis, T., Wiechmann, K., Preissner, K. T. & Herrmann, M. Staphylococcus aureus interactions with the endothelium: the role of bacterial “secretable expanded repertoire adhesive molecules” (SERAM) in disturbing host defense systems. Thromb. Haemost. 94 , 278–285 (2005).
Weidenmaier, C. et al. Lack of wall teichoic acids in Staphylococcus aureus leads to reduced interactions with endothelial cells and to attenuated virulence in a rabbit model of endocarditis. J. Infect. Dis. 191 , 1771–1777 (2005).
Loffler, B., Tuchscherr, L., Niemann, S. & Peters, G. Staphylococcus aureus persistence in non-professional phagocytes. Int. J. Med. Microbiol. 304 , 170–176 (2014).
Thomer, L., Schneewind, O. & Missiakas, D. Pathogenesis of Staphylococcus aureus bloodstream infections. Annu. Rev. Pathol. 11 , 343–364 (2016).
Le, K. Y. & Otto, M. Quorum-sensing regulation in staphylococci-an overview. Front. Microbiol. 6 , 1174 (2015).
Cheung, G. Y., Wang, R., Khan, B. A., Sturdevant, D. E. & Otto, M. Role of the accessory gene regulator agr in community-associated methicillin-resistant Staphylococcus aureus pathogenesis. Infect. Immun. 79 , 1927–1935 (2011).
Kaito, C. et al. Mobile genetic element SCCmec-encoded psm-mec RNA suppresses translation of agrA and attenuates MRSA virulence. PLoS Pathog. 9 , e1003269 (2013).
Painter, K. L., Krishna, A., Wigneshweraraj, S. & Edwards, A. M. What role does the quorum-sensing accessory gene regulator system play during Staphylococcus aureus bacteremia? Trends Microbiol. 22 , 676–685 (2014).
Crisostomo, M. I. et al. The evolution of methicillin resistance in Staphylococcus aureus: similarity of genetic backgrounds in historically early methicillin-susceptible and -resistant isolates and contemporary epidemic clones. Proc. Natl Acad. Sci. USA 98 , 9865–9870 (2001).
International Working Group on the Classification of Staphylococcal Cassette Chromosome Elements (IWG-SCC). Classification of staphylococcal cassette chromosome mec (SCCmec): guidelines for reporting novel SCCmec elements. Antimicrob. Agents Chemother. 53 , 4961–4967 (2009). This paper describes the main SCCmec types and the nomenclature to be followed for the naming of new SCC mec types.
Bal, A. M. et al. Genomic insights into the emergence and spread of international clones of healthcare-, community- and livestock-associated meticillin-resistant Staphylococcus aureus: Blurring of the traditional definitions. J. Glob. Antimicrob. Resist 6 , 95–101 (2016).
Hartman, B. J. & Tomasz, A. Low-affinity penicillin-binding protein associated with beta-lactam resistance in Staphylococcus aureus. J. Bacteriol. 158 , 513–516 (1984).
Garcia-Alvarez, L. et al. Meticillin-resistant Staphylococcus aureus with a novel mecA homologue in human and bovine populations in the UK and Denmark: a descriptive study. Lancet Infect. Dis. 11 , 595–603 (2011).
Kim, C. et al. Properties of a novel PBP2A protein homolog from Staphylococcus aureus strain LGA251 and its contribution to the beta-lactam-resistant phenotype. J. Biol. Chem. 287 , 36854–36863 (2012).
Milheirico, C., de Lencastre, H. & Tomasz, A. Full-genome sequencing identifies in the genetic background several determinants that modulate the resistance phenotype in methicillin-resistant Staphylococcus aureus strains carrying the novel mecC gene. Antimicrob. Agents Chemother. 61 , e02500–16 (2017).
Becker, K. et al. Plasmid-encoded transferable mecB-mediated methicillin resistance in Staphylococcus aureus. Emerg. Infect. Dis. 24 , 242–248 (2018).
Hiramatsu, K., Asada, K., Suzuki, E., Okonogi, K. & Yokota, T. Molecular cloning and nucleotide sequence determination of the regulator region of mecA gene in methicillin-resistant Staphylococcus aureus (MRSA). FEBSLett. 298 , 133–136 (1992).
Arede, P., Milheirico, C., de Lencastre, H. & Oliveira, D. C. The anti-repressor MecR2 promotes the proteolysis of the mecA repressor and enables optimal expression of beta-lactam resistance in MRSA. PLoS Pathog. 8 , e1002816 (2012).
Zhang, H. Z., Hackbarth, C. J., Chansky, K. M. & ≈Chambers, H. F. A proteolytic transmembrane signaling pathway and resistance to beta-lactams in staphylococci. Science 291 , 1962–1965 (2001).
De Lencastre, H. et al. Antibiotic resistance as a stress response: complete sequencing of a large number of chromosomal loci in Staphylococcus aureus strain COL that impact on the expression of resistance to methicillin. Microb. Drug Resist 5 , 163–175 (1999).
Kim, C. et al. The mechanism of heterogeneous beta-lactam resistance in MRSA: key role of the stringent stress response. PLoS ONE 8 , e82814 (2013).
Boyle-Vavra, S., Yin, S. & Daum, R. S. The VraS/VraR two-component regulatory system required for oxacillin resistance in community-acquired methicillin-resistant Staphylococcus aureus. FEMS Microbiol. Lett. 262 , 163–171 (2006).
Jousselin, A. et al. The Staphylococcus aureus chaperone PrsA is a new auxiliary factor of oxacillin resistance affecting penicillin-binding protein 2A. Antimicrob. Agents Chemother 60 , 1656–1666 (2015).
Kim, C. K., Milheirico, C., de Lencastre, H. & Tomasz, A. Antibiotic resistance as a stress response: recovery of high-level oxacillin resistance in methicillin-resistant Staphylococcus aureus “Auxiliary” (fem) mutants by induction of the stringent stress response. Antimicrob. Agents Chemother 61 , e00313-17 (2017).
Gardete, S. & Tomasz, A. Mechanisms of vancomycin resistance in Staphylococcus aureus. J. Clin. Invest. 124 , 2836–2840 (2014). This is a comprehensive review of the mechanisms of vancomycin resistance in S. aureus.
Klevens, R. M. et al. Invasive methicillin-resistant Staphylococcus aureus infections in the United States. JAMA 298 , 1763–1771 (2007).
Epstein, L. et al. Risk factors for invasive methicillin-resistant Staphylococcus aureus infection after recent discharge from an acute-care hospitalization, 2011–2013. Clin. Infect. Dis. 62 , 45–52 (2016).
Davis, K. A., Stewart, J. J., Crouch, H. K., Florez, C. E. & Hospenthal, D. R. Methicillin-resistant Staphylococcus aureus (MRSA) nares colonization at hospital admission and its effect on subsequent MRSA infection. Clin. Infect. Dis. 39 , 776–782 (2004).
Francis, J. S. et al. Severe community-onset pneumonia in healthy adults caused by methicillin-resistant Staphylococcus aureus carrying the Panton–Valentine leukocidin genes. Clin. Infect. Dis. 40 , 100–107 (2005).
Miller, L. G. et al. Necrotizing fasciitis caused by community-associated methicillin-resistant Staphylococcus aureus in Los Angeles. N. Engl. J. Med. 352 , 1445–1453 (2005).
Kazakova, S. V. et al. A clone of methicillin-resistant Staphylococcus aureus among professional football players. N. Engl. J. Med. 352 , 468–475 (2005).
Aiello, A. E., Lowy, F. D., Wright, L. N. & Larson, E. L. Meticillin-resistant Staphylococcus aureus among US prisoners and military personnel: review and recommendations for future studies. Lancet Infect. Dis. 6 , 335–341 (2006).
Drougka, E. et al. A 12-year survey of methicillin-resistant Staphylococcus aureus infections in Greece: ST80-IV epidemic? Clin. Microbiol. Infect. 20 , O796–803 (2014).
Clinical and Laboratory Standards Institute. M100-S23. Performance Standards for Antimicrobial Susceptibility Testing; Twenty-Third Informational Supplement (CLSI, 2013).
Ryffel, C., Strassle, A., Kayser, F. H. & Berger-Bachi, B. Mechanisms of heteroresistance in methicillin-resistant Staphylococcus aureus. Antimicrob. Agents Chemother. 38 , 724–728 (1994).
Penn, C. et al. Wound infections caused by inducible meticillin-resistant Staphylococcus aureus strains. J. Glob. Antimicrob. Resist 1 , 79–83 (2013).
Winstanley, T. & Courvalin, P. Expert systems in clinical microbiology. Clin. Microbiol. Rev. 24 , 515–556 (2011). This reference is a good overview of automated systems for the detection of resistance mechanisms with a critical review of published evaluations of the performance of each system.
Bhowmick, T. et al. Controlled multicenter evaluation of a bacteriophage-based method for rapid detection of Staphylococcus aureus in positive blood cultures. J. Clin. Microbiol. 51 , 1226–1230 (2013).
Opota, O., Croxatto, A., Prod’hom, G. & Greub, G. Blood culture-based diagnosis of bacteraemia: state of the art. Clin. Microbiol. Infect. 21 , 313–322 (2015).
Romero-Gomez, M. P., Cendejas-Bueno, E., Garcia Rodriguez, J. & Mingorance, J. Impact of rapid diagnosis of Staphylococcus aureus bacteremia from positive blood cultures on patient management. Eur. J. Clin. Microbiol. Infect. Dis. 36 , 2469–2473 (2017).
Malhotra-Kumar, S. et al. Current trends in rapid diagnostics for methicillin-resistant Staphylococcus aureus and glycopeptide-resistant enterococcus species. J. Clin. Microbiol. 46 , 1577–1587 (2008).
Malhotra- Kumar, S. et al. Evaluation of molecular assays for rapid detection of methicillin-resistant Staphylococcus aureus. J. Clin. Microbiol. 48 , 4598–4601 (2010).
Blaschke, A. J. et al. Rapid identification of pathogens from positive blood cultures by multiplex polymerase chain reaction using the FilmArray system. Diagnost. Microbiol. Infect. Dis. 74 , 349–355 (2012).
Almuhayawi, M., Altun, O., Stralin, K. & Ozenci, V. Identification of microorganisms by FilmArray and matrix-assisted laser desorption ionization-time of flight mass spectrometry prior to positivity in the blood culture system. J. Clin. Microbiol. 52 , 3230–3236 (2014).
Cao, M. D. et al. Streaming algorithms for identification of pathogens and antibiotic resistance potential from real-time MinION(TM) sequencing. GigaScience 5 , 32 (2016).
Aanensen, D. M. et al. Whole-genome sequencing for routine pathogen surveillance in public health: a population snapshot of invasive Staphylococcus aureus in Europe. mBio 7 , e00444-16 (2016).
Koser, C. U. et al. Rapid whole-genome sequencing for investigation of a neonatal MRSA outbreak. N. Engl. J. Med. 366 , 2267–2275 (2012).
Millar, E. V. et al. Genomic characterization of USA300 methicillin-resistant Staphylococcus aureus (MRSA) to evaluate intraclass transmission and recurrence of skin and soft tissue infection (SSTI) among high-risk military trainees. Clin. Infect. Dis. 65 , 461–468 (2017).
Merlino, J., Leroi, M., Bradbury, R., Veal, D. & Harbour, C. New chromogenic identification and detection of Staphylococcus aureus and methicillin-resistant S. aureus . J. Clin. Microbiol. 38 , 2378–2380 (2000).
Perry, J. D. A. Decade of development of chromogenic culture media for clinical microbiology in an era of molecular diagnostics. Clin. Microbiol. Rev. 30 , 449–479 (2017). Tqhis is a comprehensive reference reviewing rapid culture methods for the detection of MRSA and other multidrug-resistant organisms.
Gazin, M. et al. Culture-based detection of methicillin-resistant Staphylococcus aureus by a network of European laboratories: an external quality assessment study. Eur. J. Clin. Microbiol. Infect. Dis. 31 , 1765–1770 (2012).
Luthje, P. et al. Identification of microorganisms grown on chromogenic media by MALDI-TOF MS. J. Microbiol. Methods 136 , 17–20 (2017).
Faron, M. L. et al. Automated scoring of chromogenic media for detection of methicillin-resistant Staphylococcus aureus by use of WASPLab image analysis software. J. Clin. Microbiol. 54 , 620–624 (2016).
Cunningham, R. et al. Effect on MRSA transmission of rapid PCR testing of patients admitted to critical care. J. Hosp. Infect. 65 , 24–28 (2007).
Derde, L. P. G. et al. Interventions to reduce colonisation and transmission of antimicrobial-resistant bacteria in intensive care units: an interrupted time series study and cluster randomised trial. Lancet Infect. Dis. 14 , 31–39 (2014).
Wu, P. J., Jeyaratnam, D., Tosas, O., Cooper, B. S. & French, G. L. Point-of-care universal screening for meticillin-resistant Staphylococcus aureus: a cluster-randomized cross-over trial. J. Hosp. Infect. 95 , 245–252 (2017).
Calfee, D. P. et al. Strategies to prevent methicillin-resistant Staphylococcus aureus transmission and infection in acute care hospitals: 2014 update. Infect. Control Hosp. Epidemiol. 35 , 772–796 (2014). This paper is an expert guidance document, with grading of the quality of the evidence, for MRSA control in acute care facilities.
World Health Organization. WHO Guidelines on Hand Hygiene in Health Care. World Alliance for Patient Safety (WHO Press, Geneva, 2009).
Sax, H. et al. ‘My five moments for hand hygiene’: a user-centred design approach to understand, train, monitor and report hand hygiene. J. Hosp. Infect. 67 , 9–21 (2007).
Pittet, D. et al. Effectiveness of a hospital-wide programme to improve compliance with hand hygiene. Lancet 356 , 1307–1312 (2000).
Stone, S. P. et al. Evaluation of the national Cleanyourhands campaign to reduce Staphylococcus aureus bacteraemia and Clostridium difficile infection in hospitals in England and Wales by improved hand hygiene: four year, prospective, ecological, interrupted time series study. BMJ 344 , e3005 (2012).
Salgado, C. D. & Farr, B. M. What proportion of hospital patients colonized with methicillin-resistant Staphylococcus aureus are identified by clinical microbiological cultures? Infect. Control Hosp. Epidemiol. 27 , 116–121 (2006).
Robicsek, A. et al. Universal surveillance for methicillin-resistant Staphylococcus aureus in 3 affiliated hospitals. Ann. Intern. Med. 148 , 409–418 (2008).
Harbarth, S. et al. Universal screening for methicillin-resistant Staphylococcus aureus at hospital admission and nosocomial infection in surgical patients. JAMA 299 , 1149–1157 (2008).
Lee, A. S. et al. Comparison of strategies to reduce meticillin-resistant Staphylococcus aureus rates in surgical patients: a controlled multicentre intervention trial. BMJ Open 3 , e003126 (2013).
Robotham, J. V. et al. Cost-effectiveness of national mandatory screening of all admissions to English National Health Service hospitals for meticillin-resistant Staphylococcus aureus: a mathematical modelling study. Lancet Infect. Dis. 16 , 348–356 (2016).
Huskins, W. C. et al. Intervention to reduce transmission of resistant bacteria in intensive care. N. Engl. J. Med. 364 , 1407–1418 (2011).
Derde, L. P. et al. Interventions to reduce colonisation and transmission of antimicrobial-resistant bacteria in intensive care units: an interrupted time series study and cluster randomised trial. Lancet Infect. Dis. 14 , 31–39 (2014).
Harris, A. D. et al. Universal glove and gown use and acquisition of antibiotic-resistant bacteria in the ICU: a randomized trial. JAMA 310 , 1571–1580 (2013).
Cepeda, J. A. et al. Isolation of patients in single rooms or cohorts to reduce spread of MRSA in intensive-care units: prospective two-centre study. Lancet 365 , 295–304 (2005).
Fatkenheuer, G., Hirschel, B. & Harbarth, S. Screening and isolation to control meticillin-resistant Staphylococcus aureus: sense, nonsense, and evidence. Lancet 385 , 1146–1149 (2015). This commentary provides an update regarding the growing evidence for and against the role of active surveillance in MRSA control.
Loeb, M., Main, C., Walker-Dilks, C. & Eady, A. Antimicrobial drugs for treating methicillin-resistant Staphylococcus aureus colonization. Cochrane Database Syst. Rev. 4 , CD003340 (2003).
Poovelikunnel, T., Gethin, G. & Humphreys, H. Mupirocin resistance: clinical implications and potential alternatives for the eradication of MRSA. J. Antimicrob. Chemother. 70 , 2681–2692 (2015).
Landelle, C. et al. Randomized, placebo-controlled, double-blind clinical trial to evaluate the efficacy of polyhexanide for topical decolonization of MRSA carriers. J. Antimicrob. Chemother. 71 , 531–538 (2016).
Bode, L. G. et al. Preventing surgical-site infections in nasal carriers of Staphylococcus aureus. N. Engl. J. Med. 362 , 9–17 (2010).
van Rijen, M. M., Bode, L. G., Baak, D. A., Kluytmans, J. A. & Vos, M. C. Reduced costs for Staphylococcus aureus carriers treated prophylactically with mupirocin and chlorhexidine in cardiothoracic and orthopaedic surgery. PLoS ONE 7 , e43065 (2012).
Huang, S. S. et al. Targeted versus universal decolonization to prevent ICU infection. N. Engl. J. Med. 368 , 2255–2265 (2013).
Ammerlaan, H. S. et al. Eradication of carriage with methicillin-resistant Staphylococcus aureus: effectiveness of a national guideline. J. Antimicrob. Chemother. 66 , 2409–2417 (2011).
Ammerlaan, H. S. et al. Eradication of carriage with methicillin-resistant Staphylococcus aureus: determinants of treatment failure. J. Antimicrob. Chemother. 66 , 2418–2424 (2011).
Talan, D. A. et al. Trimethoprim-sulfamethoxazole versus placebo for uncomplicated skin abscess. N. Engl. J. Med. 374 , 823–832 (2016).
Daum, R. S. et al. A placebo-controlled trial of antibiotics for smaller skin abscesses. N. Engl. J. Med. 376 , 2545–2555 (2017).
Liu, C. et al. Clinical practice guidelines by the Infectious Diseases Society of America for the treatment of methicillin-resistant Staphylococcus aureus infections in adults and children. Clin. Infect. Dis. 52 , e18–55 (2011). This paper represents an essential and up-to-date guidance document for management of MRSA infection.
Gould, I. M. et al. New insights into meticillin-resistant Staphylococcus aureus (MRSA) pathogenesis, treatment and resistance. Int. J. Antimicrob. Agents 39 , 96–104 (2012).
Svetitsky, S., Leibovici, L. & Paul, M. Comparative efficacy and safety of vancomycin versus teicoplanin: systematic review and meta-analysis. Antimicrob. Agents Chemother. 53 , 4069–4079 (2009).
Vandecasteele, S. J., De Vriese, A. S. & Tacconelli, E. The pharmacokinetics and pharmacodynamics of vancomycin in clinical practice: evidence and uncertainties. J. Antimicrob. Chemother. 68 , 743–748 (2013).
Lodise, T. P., Patel, N., Lomaestro, B. M., Rodvold, K. A. & Drusano, G. L. Relationship between initial vancomycin concentration-time profile and nephrotoxicity among hospitalized patients. Clin. Infect. Dis. 49 , 507–514 (2009).
Cataldo, M. A., Tacconelli, E., Grilli, E., Pea, F. & Petrosillo, N. Continuous versus intermittent infusion of vancomycin for the treatment of Gram-positive infections: systematic review and meta-analysis. J. Antimicrob. Chemother. 67 , 17–24 (2012).
Bradley, J. S. Which antibiotic for resistant Gram-positives, and why? J. Infect. 68 (Suppl. 1), S63–75 (2014).
Falagas, M. E. & Vardakas, K. Z. Benefit-risk assessment of linezolid for serious gram-positive bacterial infections. DrugSaf. 31 , 753–768 (2008).
Cosgrove, S. E. et al. Comparison of mortality associated with methicillin-resistant and methicillin-susceptible Staphylococcus aureus bacteremia: a meta-analysis. Clin. Infect. Dis. 36 , 53–59 (2003).
Fowler, V. G. Jr. et al. Clinical identifiers of complicated Staphylococcus aureus bacteremia. Arch. Intern. Med. 163 , 2066–2072 (2003).
Thwaites, G. E. et al. Clinical management of Staphylococcus aureus bacteraemia. Lancet Infect. Dis. 11 , 208–222 (2011). This is an important reference for all persons interested in the optimal treatment of S. aureus bacteraemia.
Houlihan, H. H., Mercier, R. C. & Rybak, M. J. Pharmacodynamics of vancomycin alone and in combination with gentamicin at various dosing intervals against methicillin-resistant Staphylococcus aureus-infected fibrin-platelet clots in an in vitro infection model. Antimicrob. Agents Chemother. 41 , 2497–2501 (1997).
Tsuji, B. T. & Rybak, M. J. Short-course gentamicin in combination with daptomycin or vancomycin against Staphylococcus aureus in an in vitro pharmacodynamic model with simulated endocardial vegetations. Antimicrob. Agents Chemother. 49 , 2735–2745 (2005).
Rehm, S. J. et al. Daptomycin versus vancomycin plus gentamicin for treatment of bacteraemia and endocarditis due to Staphylococcus aureus: subset analysis of patients infected with methicillin-resistant isolates. J. Antimicrob. Chemother. 62 , 1413–1421 (2008).
Fowler, V. G. Jr. et al. Daptomycin versus standard therapy for bacteremia and endocarditis caused by Staphylococcus aureus. N. Engl. J. Med. 355 , 653–665 (2006).
Bayer, A. S. & Murray, B. E. Initial low-dose aminoglycosides in Staphylococcus aureus bacteremia: good science, urban legend, or just plain toxic? Clin. Infect. Dis. 48 , 722–724 (2009).
Forrest, G. N. & Tamura, K. Rifampin combination therapy for nonmycobacterial infections. Clin. Microbiol. Rev. 23 , 14–34 (2010).
Thwaites, G. E. et al. Adjunctive rifampicin for Staphylococcus aureus bacteraemia (ARREST): a multicentre, randomised, double-blind, placebo-controlled trial. Lancet 391 , 668–678 (2018).
Davis, J. S. et al. Combination of vancomycin and beta-lactam therapy for methicillin-resistant Staphylococcus aureus bacteremia: a pilot multicenter randomized controlled trial. Clin. Infect. Dis. 62 , 173–180 (2016).
Sakoulas, G. et al. Antimicrobial salvage therapy for persistent staphylococcal bacteremia using daptomycin plus ceftaroline. Clin. Ther. 36 , 1317–1333 (2014).
Rose, W. E., Berti, A. D., Hatch, J. B. & Maki, D. G. Relationship of in vitro synergy and treatment outcome with daptomycin plus rifampin in patients with invasive methicillin-resistant Staphylococcus aureus infections. Antimicrob. Agents Chemother. 57 , 3450–3452 (2013).
Cosimi, R. A., Beik, N., Kubiak, D. W. & Johnson, J. A. Ceftaroline for severe methicillin-resistant Staphylococcus aureus infections: a systematic review. Open Forum Infect. Dis. 4 , ofx084 (2017).
Tattevin, P., Basuino, L., Bauer, D., Diep, B. A. & Chambers, H. F. Ceftobiprole is superior to vancomycin, daptomycin, and linezolid for treatment of experimental endocarditis in rabbits caused by methicillin-resistant Staphylococcus aureus. Antimicrob. Agents Chemother. 54 , 610–613 (2010).
Oltolini, C. et al. Meticillin-resistant Staphylococcus aureus endocarditis: first report of daptomycin plus ceftobiprole combination as salvage therapy. Int. J. Antimicrob. Agents 47 , 502–504 (2016).
Andrey, D. O. et al. Antimicrobial activity of ceftaroline against methicillin-resistant Staphylococcus aureus (MRSA) isolates collected in 2013–2014 at the Geneva University Hospitals. Eur. J. Clin. Microbiol. Infect. Dis. 36 , 343–350 (2017).
Long, S. W. et al. PBP2a mutations causing high-level Ceftaroline resistance in clinical methicillin-resistant Staphylococcus aureus isolates. Antimicrob. Agents Chemother. 58 , 6668–6674 (2014).
Mendes, R. E. et al. Characterization of methicillin-resistant Staphylococcus aureus displaying increased MICs of ceftaroline. J. Antimicrob. Chemother. 67 , 1321–1324 (2012).
Zhanel, G. G. et al. New lipoglycopeptides: a comparative review of dalbavancin, oritavancin and telavancin. Drugs 70 , 859–886 (2010).
McCurdy, S. P., Jones, R. N., Mendes, R. E., Puttagunta, S. & Dunne, M. W. In vitro activity of dalbavancin against drug-resistant Staphylococcus aureus isolates from a global surveillance program. Antimicrob. Agents Chemother. 59 , 5007–5009 (2015).
Werth, B. J. et al. Emergence of dalbavancin non-susceptible, vancomycin-intermediate Staphylococcus aureus (VISA) after treatment of MRSA central line-associated bloodstream infection with a dalbavancin- and vancomycin-containing regimen. Clin. Microbiol. Infect. 24 , 429.e1–429.e5 (2018).
Yaw, L. K., Robinson, J. O. & Ho, K. M. A comparison of long-term outcomes after meticillin-resistant and meticillin-sensitive Staphylococcus aureus bacteraemia: an observational cohort study. Lancet Infect. Dis. 14 , 967–975 (2014).
Fatkenheuer, G. & Kaasch, A. J. How deadly is meticillin-resistant Staphylococcus aureus? Lancet Infect. Dis. 14 , 905–907 (2014).
De Angelis, G. et al. Multistate modelling to estimate the excess length of stay associated with meticillin-resistant Staphylococcus aureus colonisation and infection in surgical patients. J. Hosp. Infect. 78 , 86–91 (2011).
De Angelis, G., Murthy, A., Beyersmann, J. & Harbarth, S. Estimating the impact of healthcare-associated infections on length of stay and costs. Clin. Microbiol. Infect. 16 , 1729–1735 (2010).
Stewardson, A. J. et al. The health and economic burden of bloodstream infections caused by antimicrobial-susceptible and non-susceptible Enterobacteriaceae and Staphylococcus aureus in European hospitals, 2010 and 2011: a multicentre retrospective cohort study. Eurosurveillance 21 , 30319 (2016). This is a methodologically sound analysis of excess length of stay of MRSA bacteraemia.
Haessler, S., Mackenzie, T. & Kirkland, K. B. Long-term outcomes following infection with meticillin-resistant or meticillin-susceptible Staphylococcus aureus. J. Hosp. Infect. 69 , 39–45 (2008).
Pendleton, A. & Kocher, M. S. Methicillin-resistant Staphylococcus aureus bone and joint infections in children. J. Am. Acad. Orthop. Surg. 23 , 29–37 (2015).
Salgado, C. D., Dash, S., Cantey, J. R. & Marculescu, C. E. Higher risk of failure of methicillin-resistant Staphylococcus aureus prosthetic joint infections. Clin. Orthop. Relat. Res. 461 , 48–53 (2007).
Gleeson, A., Larkin, P. & O'Sullivan, N. The impact of meticillin-resistant Staphylococcus aureus on patients with advanced cancer and their family members: a qualitative study. Palliat. Med. 30 , 382–391 (2016).
van Cleef, B. A. et al. Health and health-related quality of life in pig farmers carrying livestock-associated methicillin-resistant Staphylococcus aureus. Epidemiol. Infect. 144 , 1774–1783 (2016).
Ammerlaan, H. S. et al. Secular trends in nosocomial bloodstream infections: antibiotic-resistant bacteria increase the total burden of infection. Clin. Infect. Dis. 56 , 798–805 (2013). This is most likely the best paper evaluating the question of whether MRSA adds to the overall burden of nosocomial infections.
Jones, B. E. et al. Variation in empiric coverage versus detection of methicillin-resistant Staphylococcus aureus and Pseudomonas aeruginosa in hospitalizations for community-onset pneumonia across 128 US veterans affairs medical centers. Infect. Control Hosp. Epidemiol. 38 , 937–944 (2017).
Herr, C. E., Heckrodt, T. H., Hofmann, F. A., Schnettler, R. & Eikmann, T. F. Additional costs for preventing the spread of methicillin-resistant Staphylococcus aureus and a strategy for reducing these costs on a surgical ward. Infect. Control Hosp. Epidemiol. 24 , 673–678 (2003).
Wertheim, H. F. et al. Risk and outcome of nosocomial Staphylococcus aureus bacteraemia in nasal carriers versus non-carriers. Lancet 364 , 703–705 (2004).
Pozzi, C. et al. Vaccines for Staphylococcus aureus and target populations. Curr. Top. Microbiol. Immunol. 409 , 491–528 (2017).
Fowler, V. G. et al. Effect of an investigational vaccine for preventing Staphylococcus aureus infections after cardiothoracic surgery: a randomized trial. JAMA 309 , 1368–1378 (2013).
Shinefield, H. et al. Use of a Staphylococcus aureus conjugate vaccine in patients receiving hemodialysis. N. Engl. J. Med. 346 , 491–496 (2002).
Fowler, V. G. Jr & Proctor, R. A. Where does a Staphylococcus aureus vaccine stand? Clin. Microbiol. Infect. 20 (Suppl. 5), 66–75 (2014).
Stentzel, S. et al. Specific serum IgG at diagnosis of Staphylococcus aureus bloodstream invasion is correlated with disease progression. J. Proteom. 128 , 1–7 (2015).
Kurokawa, K., Takahashi, K. & Lee, B. L. The staphylococcal surface-glycopolymer wall teichoic acid (WTA) is crucial for complement activation and immunological defense against Staphylococcus aureus infection. Immunobiology 221 , 1091–1101 (2016).
Bekeredjian-Ding, I. Deciphering the significance of the T cell response to Staphylococcus aureus. Future Microbiol. 12 , 1023–1026 (2017).
Lehar, S. M. et al. Novel antibody-antibiotic conjugate eliminates intracellular S. aureus . Nature 527 , 323–328 (2015).
World Health Organization. Prioritization of Pathogens to Guide Discovery, Research and Development of New Antibiotics for Drug-Resistant Bacterial Infections, Including Tuberculosis (WHO, Geneva, 2017).
Idelevich, E. A. et al. In vitro activity against Staphylococcus aureus of a novel antimicrobial agent, PRF-119, a recombinant chimeric bacteriophage endolysin. Antimicrob. Agents Chemother. 55 , 4416–4419 (2011).
Hiramatsu, K. et al. Methicillin-resistant Staphylococcus aureus clinical strain with reduced vancomycin susceptibility. J. Antimicrob. Chemother. 40 , 135–136 (1997).
Mwangi, M. M. et al. Tracking the in vivo evolution of multidrug resistance in Staphylococcus aureus by whole-genome sequencing. Proc. Natl Acad. Sci. USA 104 , 9451–9456 (2007).
Howden, B. P., Davies, J. K., Johnson, P. D., Stinear, T. P. & Grayson, M. L. Reduced vancomycin susceptibility in Staphylococcus aureus, including vancomycin-intermediate and heterogeneous vancomycin-intermediate strains: resistance mechanisms, laboratory detection, and clinical implications. Clin. Microbiol. Rev. 23 , 99–139 (2010).
Khatib, R. et al. Relevance of vancomycin-intermediate susceptibility and heteroresistance in methicillin-resistant Staphylococcus aureus bacteraemia. J. Antimicrob. Chemother. 66 , 1594–1599 (2011).
Satola, S. W. et al. Clinical and laboratory characteristics of invasive infections due to methicillin-resistant Staphylococcus aureus isolates demonstrating a vancomycin MIC of 2 micrograms per milliliter: lack of effect of heteroresistant vancomycin-intermediate S. aureus phenotype. J. Clin. Microbiol. 49 , 1583–1587 (2011).
Sievert, D. M. et al. Vancomycin-resistant Staphylococcus aureus in the United States, 2002–2006. Clin. Infect. Dis. 46 , 668–674 (2008).
Weigel, L. M. et al. Genetic analysis of a high-level vancomycin-resistant isolate of Staphylococcus aureus. Science 302 , 1569–1571 (2003).
Foucault, M. L., Courvalin, P. & Grillot-Courvalin, C. Fitness cost of VanA-type vancomycin resistance in methicillin-resistant Staphylococcus aureus. Antimicrob. Agents Chemother. 53 , 2354–2359 (2009).
Stefani, S. et al. Meticillin-resistant Staphylococcus aureus (MRSA): global epidemiology and harmonisation of typing methods. Int. J. Antimicrob. Agents 39 , 273–282 (2012).
Carlet, J. et al. French national program for prevention of healthcare-associated infections and antimicrobial resistance, 1992-2008: positive trends, but perseverance needed. Infect. Control Hosp. Epidemiol. 30 , 737–745 (2009).
Duerden, B., Fry, C., Johnson, A. P. & Wilcox, M. H. The control of methicillin-resistant Staphylococcus aureus blood stream infections in England. Open Forum Infect. Dis. 2 , ofv035 (2015).
[No authors listed.] PubMLST. University of Oxford https://pubmlst.org/ (2018).
Thurlow, L. R., Joshi, G. S. & Richardson, A. R. Virulence strategies of the dominant USA300 lineage of community-associated methicillin-resistant Staphylococcus aureus (CA-MRSA). FEMS Immunol. Med. Microbiol. 65 , 5–22 (2012).
Peschel, A. & Otto, M. Phenol-soluble modulins and staphylococcal infection. Nat. Rev. Microbiol. 11 , 667–673 (2013).
Wright, G. D. Q&A: Antibiotic resistance: where does it come from and what can we do about it? BMC Biol. 8 , 123 (2010).
McCarthy, A. J. & Lindsay, J. A. Staphylococcus aureus innate immune evasion is lineage-specific: a bioinfomatics study. Infect. Genet. Evol. 19 , 7–14 (2013).
Markowitz, N., Quinn, E. L. & Saravolatz, L. D. Trimethoprim-sulfamethoxazole compared with vancomycin for the treatment of Staphylococcus aureus infection. Ann. Intern. Med. 117 , 390–398 (1992).
Landersdorfer, C. B., Bulitta, J. B., Kinzig, M., Holzgrabe, U. & Sorgel, F. Penetration of antibacterials into bone: pharmacokinetic, pharmacodynamic and bioanalytical considerations. Clin. Pharmacokinet. 48 , 89–124 (2009).
Sakoulas, G., Alder, J., Thauvin-Eliopoulos, C., Moellering, R. C. Jr & Eliopoulos, G. M. Induction of daptomycin heterogeneous susceptibility in Staphylococcus aureus by exposure to vancomycin. Antimicrob. Agents Chemother. 50 , 1581–1585 (2006).
Pertel, P. E. et al. Effects of prior effective therapy on the efficacy of daptomycin and ceftriaxone for the treatment of community-acquired pneumonia. Clin. Infect. Dis. 46 , 1142–1151 (2008).
Fenton, C., Keating, G. M. & Curran, M. P. Daptomycin. Drugs 64 , 445–455 (2004).
Pea, F. et al. Therapeutic drug monitoring may improve safety outcomes of long-term treatment with linezolid in adult patients. J. Antimicrob. Chemother. 67 , 2034–2042 (2012).
Itani, K. M. et al. Efficacy and safety of linezolid versus vancomycin for the treatment of complicated skin and soft-tissue infections proven to be caused by methicillin-resistant Staphylococcus aureus. Am. J. Surg. 199 , 804–816 (2010).
Corey, G. R. et al. CANVAS 1: the first Phase III, randomized, double-blind study evaluating ceftaroline fosamil for the treatment of patients with complicated skin and skin structure infections. J. Antimicrob. Chemother. 65 (Suppl. 4), iv41–iv51 (2010).
Wilcox, M. H. et al. CANVAS 2: the second Phase III, randomized, double-blind study evaluating ceftaroline fosamil for the treatment of patients with complicated skin and skin structure infections. J. Antimicrob. Chemother. 65 (Suppl. 4), iv53–iv65 (2010).
Dryden, M., Zhang, Y., Wilson, D., Iaconis, J. P. & Gonzalez, J. A. Phase III, randomized, controlled, non-inferiority trial of ceftaroline fosamil 600 mg every 8 h versus vancomycin plus aztreonam in patients with complicated skin and soft tissue infection with systemic inflammatory response or underlying comorbidities. J. Antimicrob. Chemother. 71 , 3575–3584 (2016).
Noel, G. J., Bush, K., Bagchi, P., Ianus, J. & Strauss, R. S. A randomized, double-blind trial comparing ceftobiprole medocaril with vancomycin plus ceftazidime for the treatment of patients with complicated skin and skin-structure infections. Clin. Infect. Dis. 46 , 647–655 (2008).
[No authors listed.] Vibativ (telavancin) [prescribing information]. Astellas http://www.astellas.us/docs/us/VIBATIV_PI_Final.pdf (2009).
Rubinstein, E. et al. Telavancin versus vancomycin for hospital-acquired pneumonia due to gram-positive pathogens. Clin. Infect. Dis. 52 , 31–40 (2011).
Stryjewski, M. E. et al. A randomized Phase 2 trial of telavancin versus standard therapy in patients with uncomplicated Staphylococcus aureus bacteremia: the ASSURE study. BMC Infect. Dis. 14 , 289 (2014).
Nord, C. E., Rasmanis, G. & Wahlund, E. Effect of dalbavancin on the normal intestinal microflora. J. Antimicrob. Chemother. 58 , 627–631 (2006).
Ramdeen, S. & Boucher, H. W. Dalbavancin for the treatment of acute bacterial skin and skin structure infections. Expert Opin. Pharmacother. 16 , 2073–2081 (2015).
Messina, J. A., Fowler, V. G. Jr & Corey, G. R. Oritavancin for acute bacterial skin and skin structure infections. Expert Opin. Pharmacother. 16 , 1091–1098 (2015).
Biedenbach, D. J., Arhin, F. F., Moeck, G., Lynch, T. F. & Sahm, D. F. In vitro activity of oritavancin and comparator agents against staphylococci, streptococci and enterococci from clinical infections in Europe and North America, 2011–2014. Int. J. Antimicrob. Agents 46 , 674–681 (2015).
Prokocimer, P., De Anda, C., Fang, E., Mehra, P. & Das, A. Tedizolid phosphate versus linezolid for treatment of acute bacterial skin and skin structure infections: the ESTABLISH-1 randomized trial. JAMA 309 , 559–569 (2013).
Moran, G. J. et al. Tedizolid for 6 days versus linezolid for 10 days for acute bacterial skin and skin-structure infections (ESTABLISH-2): a randomised, double-blind, phase 3, non-inferiority trial. Lancet Infect. Dis. 14 , 696–705 (2014).
Van Bambeke, F. Delafloxacin, a non-zwitterionic fluoroquinolone in Phase III of clinical development: evaluation of its pharmacology, pharmacokinetics, pharmacodynamics and clinical efficacy. Future Microbiol. 10 , 1111–1123 (2015).
Kingsley, J. et al. A randomized, double-blind, Phase 2 study to evaluate subjective and objective outcomes in patients with acute bacterial skin and skin structure infections treated with delafloxacin, linezolid or vancomycin. J. Antimicrob. Chemother 71 , 821–829 (2016).
Pullman, J. et al. Efficacy and safety of delafloxacin compared with vancomycin plus aztreonam for acute bacterial skin and skin structure infections: a phase 3, double-blind, randomized study. J. Antimicrob. Chemother. 72 , 3471–3480 (2017).
Download references
Acknowledgements
H.d.L. thanks A. Tomasz and C. Milheiriço for helpful discussions while preparing the manuscript. H.d.L.'s research is supported by Project LISBOA-01-0145-FEDER-007660 funded by the European Regional Development Fund through COMPETE2020 (POCI) and by national funds through Fundação para a Ciência e Tecnologia. A.P. thanks S. Heilbronner for helpful discussions and critical reading of the manuscript. A.P.'s research is supported by grants from the Deutsche Forschungsgemeinschaft (TRR34, TRR156, SFB766, SFB685, GRK1708 and PE805/5-1), the Deutsches Zentrum für Infektionsforschung (TTU HAARBI) and the European Innovative Medicines Initiative (COMBACTE). S.H. receives financial support from COMBACTE.
Author information
Authors and affiliations.
Departments of Infectious Diseases and Microbiology, Royal Prince Alfred Hospital, Sydney, New South Wales, Australia
Andie S. Lee
Faculty of Medicine, University of Sydney, Sydney, New South Wales, Australia
Laboratory of Microbiology and Infectious Diseases, The Rockefeller University, New York, NY, USA
Hermínia de Lencastre
Laboratory of Molecular Genetics, Instituto de Tecnologia Química e Biológica António Xavier, Universidade Nova de Lisboa, Oeiras, Portugal
Department of Medicine, Hospital Universitari Mutua de Terrassa, Barcelona, Spain
Javier Garau
Department of Infection Control, Amphia Hospital, Breda, Netherlands
Jan Kluytmans
Julius Center for Health Sciences and Primary Care, University Medical Center Utrecht, Utrecht University, Utrecht, Netherlands
Laboratory of Medical Microbiology, Vaccine and Infectious Disease Institute, Universiteit Antwerpen, Wilrijk, Belgium
Surbhi Malhotra-Kumar
Infection Biology Department, Interfaculty Institute of Microbiology and Infection Medicine, University of Tübingen, Tübingen, Germany
Andreas Peschel
German Center for Infection Research, Partner Site Tübingen, Tübingen, Germany
Infection Control Programme, University of Geneva Hospitals and Faculty of Medicine, WHO Collaborating Center, Geneva, Switzerland
Stephan Harbarth
You can also search for this author in PubMed Google Scholar
Contributions
Introduction (A.S.L.); Epidemiology (A.S.L. and S.H.); Mechanisms/pathophysiology (H.d.L., S.M.-K., J.K. and A.P.); Diagnosis, screening and prevention (A.S.L., S.M.-K. and S.H.); Management (J.K., S.H. and J.G.); Quality of life (S.H.); Outlook (A.P. and S.H.); Overview of Primer (S.H. and A.S.L.).
Corresponding authors
Correspondence to Andie S. Lee or Stephan Harbarth .
Ethics declarations
Competing interests.
J.G. has acted as a consultant for Roche, Nabriva, Paratek and Menarini. J.K. acts as a consultant for Pfizer, 3M and Destiny Pharma. S.M.-K. has received grants from Abbott and Agfa Health. She is receiving research grants from Pfizer and Huvepharma and has a service agreement with AiCuris. A.P. receives a consultant fee from Crucell and research grants from Crucell, Medimmune, MorphoSys and Roche; he has a patent pending for lugdunin. S.H. was a temporary member of the speakers’ bureau for Takeda; has participated in the scientific advisory boards of DNA Electronics, Sandoz, GlaxoSmithKline and Bayer; and has received financial support for research activities from Pfizer and B. Braun. A.S.L. and H.d.L. declare no conflicts of interest.
PowerPoint slides
Powerpoint slide for fig. 1, powerpoint slide for fig. 2, powerpoint slide for fig. 3, powerpoint slide for fig. 4, powerpoint slide for fig. 5, rights and permissions.
Reprints and permissions
About this article
Cite this article.
Lee, A., de Lencastre, H., Garau, J. et al. Methicillin-resistant Staphylococcus aureus . Nat Rev Dis Primers 4 , 18033 (2018). https://doi.org/10.1038/nrdp.2018.33
Download citation
Published : 31 May 2018
DOI : https://doi.org/10.1038/nrdp.2018.33
Share this article
Anyone you share the following link with will be able to read this content:
Sorry, a shareable link is not currently available for this article.
Provided by the Springer Nature SharedIt content-sharing initiative
This article is cited by
Immunization with a peptide mimicking lipoteichoic acid induces memory b cells in balb/c mice.
- Xiao-Rui Hou
BMC Infectious Diseases (2024)
The role of Staphylococcus aureus quorum sensing in cutaneous and systemic infections
- Yuriko Yamazaki
- Yuumi Nakamura
Inflammation and Regeneration (2024)
Synergistic use of anti-inflammatory ketorolac and gentamicin to target staphylococcal biofilms
- Amita Sekar
Journal of Translational Medicine (2024)
Anti-biofilm properties of laser-synthesized, ultrapure silver–gold-alloy nanoparticles against Staphylococcus aureus
- Katharina Doll-Nikutta
- Meike Stiesch
Scientific Reports (2024)
Using novel micropore technology combined with artificial intelligence to differentiate Staphylococcus aureus and Staphylococcus epidermidis
- Ayumi Morimura
- Masateru Taniguchi
- Shigeto Hamaguchi
Quick links
- Explore articles by subject
- Guide to authors
- Editorial policies
Sign up for the Nature Briefing newsletter — what matters in science, free to your inbox daily.

- Open access
- Published: 30 June 2023
Methicillin-resistant Staphylococcus aureus : novel treatment approach breakthroughs
- Balaji Maddiboyina ORCID: orcid.org/0000-0001-7322-306X 1 , 5 na1 ,
- Harekrishna Roy 2 na1 ,
- M. Ramaiah 1 na1 ,
- C. N. Sarvesh 1 ,
- Sahasra Hanuman Kosuru 1 ,
- Ramya Krishna Nakkala 3 &
- Bhabani Shankar Nayak 4
Bulletin of the National Research Centre volume 47 , Article number: 95 ( 2023 ) Cite this article
5175 Accesses
1 Citations
2 Altmetric
Metrics details
Methicillin-resistant Staphylococcus aureus (MRSA) is a common bacterial infection that is a significant source of illness and mortality globally. The advancements in antibiotic therapies continue to be the critical aspects of treating bacterial infections, and their usage has lowered patient mortality and raised life expectancy.
Main body of the abstract
The ideal treatments for MRSA remain challenging, and the quest for new antibiotic targets and advanced drug delivery systems with safety profiles is necessary to ensure treating MRSA infections adequately in the future.
Short conclusion
This article primarily focuses on different therapeutic medications and their modes of action for general microbial infections and goes through the latest developments in novel drug delivery technologies, such as hydrogels, lipid particles, nanocarriers, and polymers for MRSA treatment.
Graphical Abstract
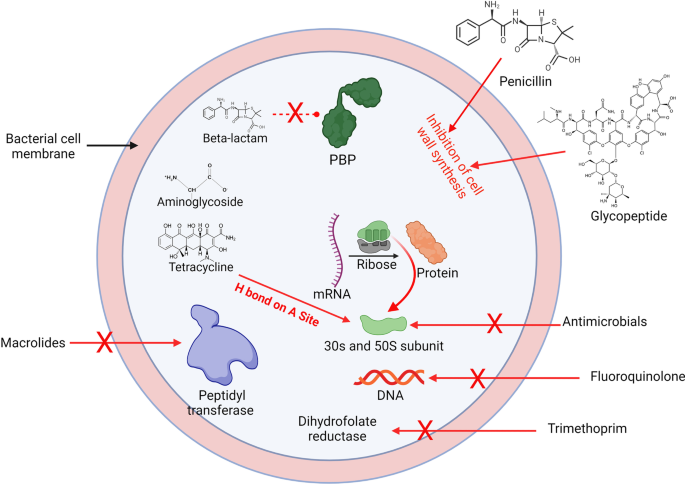
Methicillin-resistant Staphylococcus aureus (MRSA) is an infection seen in many hospitals. It is a type of bacteria that are especially prevalent in hospitals and can cause severe sickness and death (Turner et al. 2019 ). According to recent prevalence studies, the incidence of MRSA is on the rise in developed countries, both in healthcare settings and in the community (Wan et al. 2021 ; Maddina et al. 2016 ). Among nosocomial infections in developed countries, MRSA poses a 60% risk of colonization (Williams et al. 2009 ). A recent meta-analysis of inpatient admissions for S. aureus infection in the USA reported nearly 4,000,000 population admissions per year. MRSA also increases the mortality rate (~ 19,000) of the hospitalized American population daily (Report NDS 2020 ; Maddiboyina et al. 2020 ).
Staphylococcus aureus , gram-positive bacteria in the Stpohylococcceae family with a diameter of about 1 μm, produces grape-like collections. S. aureus is a bacterium that lives commensally or often symptomless in healthy individuals’ skin and skin glands, nasal epithelial cells, mucous membranes, and gut (Raineri et al. 2022 ). Several prevalence revisions revealed that 20% of populations remain obstinate nasal carriers, approximately 30% of populations are recurrent carriers, while 50% remain non-carriers. In 1880, Alexander Ogston sequestered S. aureus following a surgical wound contagion (Ogston 1882 ). It has been a long time since MRSA’s prevalence and incidence have skyrocketed worldwide. According to recent epidemiology studies, the global incidence of MRSA infection has increased by 7 to 60% between 1964 and 2015 (Cowan et al. 1954 ; Maddiboyina et al. 2023 ). Extended hospitalization, intensive care, open wounds, hemodialysis, overdosing on antibiotics, MRSA colonization, and long-term urinary catheter use are all risk factors for MRSA. People suffering from major chronic illnesses are at high risk of contracting MRSA. Detecting MRSA colonization during admission may reduce the risk of developing MRSA infections (Sakr et al. 2018 ). Increasing antibiotic resistance is a result of MRSA infection. This limits treatment options for MRSA-associated infections. As well as poor infection control, MRSA remains resistant to the utmost powerful antibiotics employed to treat severe MRSA infections, according to the latest research. Aminoglycosides, erythromycin, and fluoroquinolones are the most commonly prescribed antibiotics for MRSA treatment. Vancomycin remains the most frequently used antibiotic in the therapy of MRSA (Cong et al. 2020 ). A schematic representation of timeline for novel treatment approach and development of resistance of staphylococcus aureus is described in Fig. 1 .
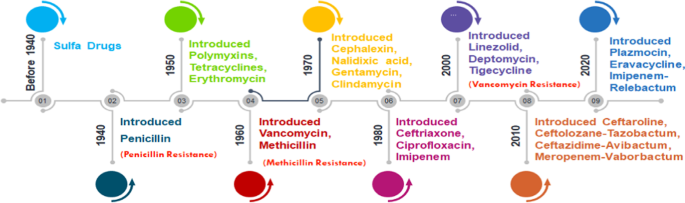
Schematic representation of timeline of antimicrobial drug development and the subsequent emergence of resistance
Mechanism of antibiotic action and antibiotics resistance
Antibiotics are classified based on their mechanism of action (MoA), as shown in Fig. 2 .
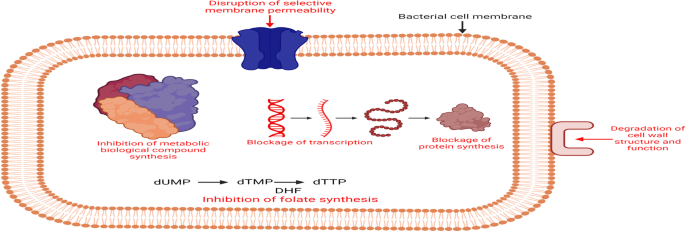
Different targets of antimicrobials treatment
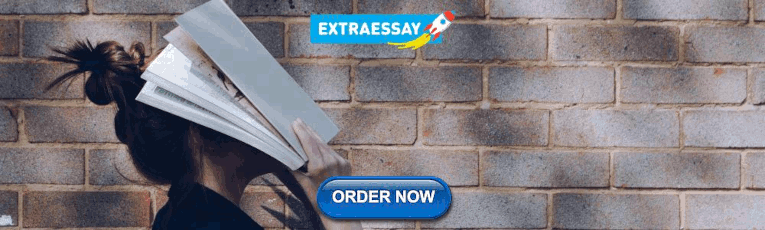
Antibiotics that attack the cell wall
This large sugar polymer, peptidoglycan, undergoes crosslinking of the glycan components due to transglycosidases, which crosslink the glycan components. In the incidence of penicillin-binding proteins, glycine deposits crosslink the D-alanine-alanine peptide chain (Maya-Martinez et al. 2019 ). Unlike β-lactams and glycopeptides, which interfere with the cell wall’s construction, β-lactams and glycopeptides do the opposite by blocking the production of the cell wall.
Beta-lactam antibiotics
PBPs continue to be the primary goal of -lactam assistance, and they will continue to be. The PBP binds to the β-lactam ring and cannot be used to produce other peptidoglycans because it interacts with the ring. In this case, disruption of the peptidoglycan stratum indicates that the bacteria are on the verge of lysing (Iyer 2022 ).
Glycopeptides
Glycopeptides bind to the peptide side chain’s d -alanyl d -alanine section in the d -alanyl d -alanine sequence. It prevents d -alanyl subsets from binding to the PBP and thus prevents cell wall synthesis (Blaskovich et al. 2018 ).
Inhibitors of protein biosynthesis
Translation occurs when the ribosome synthesizes proteins from messenger RNA (mRNA). Ribosomes and cytoplasmic factors are still involved in the protein synthesis process. Antimicrobials aim at the 30S or 50S subunits of the bacterial ribosome to inhibit protein synthesis (Roy et al. 2022 ).
Inhibitors of 30S subunit
Aminoglycosides (ags).
AGs are negatively-charged molecules that are charged positively and therefore attach to the outside of the cell’s membrane. This allows for the formation of large pores, which allows antibiotics to permeate into the bacterium and kill the bacteria. Bacteria get into the cell via cell wall synthesis; antibiotics that inhibit this process, such as β-lactams and glycopeptides, make it easier for AGs to get into cells at low doses because of the effect of those antibiotics. The AGs’ interaction with the 16S r-RNA of the 30S subunit is via a hydrogen bond, which is adjacent to the A site. The diseases cause delusions and random mutations in mRNA.
Tetracyclines
They are derived from the preserved orders of the 30S ribosomal subunit’s 16S r-RNA to prevent t-RNA binding toward the A site (Maddiboyina et al. 2022a ).
Inhibitors of 50S subunit
Chloramphenicol.
This connects via the sealed orders of the peptidyl transferase opening of the 50S subunit’s 23S r-RNA. Following that, this inhibits protein synthesis through averting t-RNA from binding toward the A site of the ribosome (Sanga et al. 2022 ).
This is accomplished by targeting the peptidyl transferase focus of the 50S ribosomal subunit’s 23S r-RNA (Zimmermann et al. 2018 ). All of these macrolides, lincosamides, and streptogramins display the same MoA.
Oxazolidinones
They work by interacting with the 50S subunit’s 23Sr RNA and by reducing the 70S inhibition and interaction via peptidyl-tRNA (Roger et al. 2018 ).
Inhibitors of DNA replication
This enzyme marks double-stranded DNA, familiarizes negative supercoils, and later seals the pilfered ends. Fluoroquinolone (FQ) inhibits this enzyme. This is important to keep excessive positive supercoiling in check after the strands have separated. Their strand-cutting/resealing abilities restrict anatomically similar FQs binding to A subunit. Prior to DNA replication, Topoisomerase IV’s nick and split process attacks and breaks up the daughter DNA strands, which are Gram-positive bacteria’s primary target. Compared to Gram-positive bacteria, this enzyme may be more effective because it is more similar. Because topoisomerase II is more closely related to FQ than DNA gyrase or topoisomerase IV, it is retained in mammalian cells (Bush et al. 2020 ).
Folic acid metabolism inhibitors
Sulfonamides and trimethoprim.
At different stages of the same biosynthesis pathway, trimethoprim is combined with sulfonamides to create interaction and compact the mutation rate for resistance. Sulfonamides inhibit DHS because they are more similar to the enzyme than their likely substrate, p-aminobenzoic acid. Inhibition of the enzyme dihydrofolate reductase by trimethoprim, for example, occurs at an advanced stage in the production of folic acid (Ovung and Bhattacharyya 2021 ).
Mechanisms of antimicrobial resistance
Blocked penetration.
Depending on the drug’s nature, drugs can be delivered to a cell via porin diffusion, bilayer diffusion, or self-uptake. It has been shown that porins can traverse the outer membrane through themselves with the help of slight hydrophilic molecules (β-lactam and quinolones). If the number of porin passages is reduced, β-lactam antibiotics and FQ obsessed are less likely to enter the cell, resulting in later resistance to these antibiotic classes. Antibiotic resistance in P. aeruginosa has developed due to its inability to penetrate the bacterium’s outer membrane as displayed in Fig. 3 .
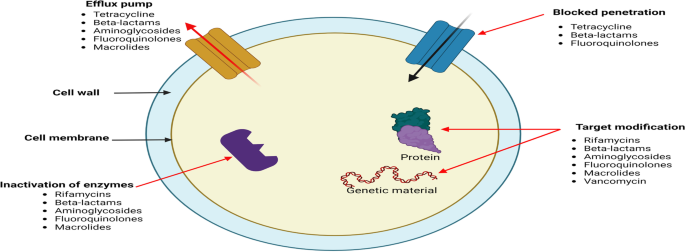
Antimicrobial drug resistance mechanisms in microorganisms
Efflux pumps
Cellular efflux mechanisms prevent the antimicrobials from reaching their intended target (Reygaert 2018 ). However, the cytoplasmic membrane still has these pumps, while the outer membrane has distinct porin proteins. In terms of antibiotics, efflux pumps can be quite specific. Because they are multidrug carriers capable of pumping multiple antibiotics such as macrolides, tetracyclines, and FQ, multidrug-resistant organisms are reduced in numbers (Maddiboyina et al. 2015 ).
Modification of target molecule
They need an imperative impact on antibiotic binding because they’re often caused by an unintentional mutation of one of the bacteria’s genes on the genome. By interfering with protein synthesis, alterations in the 30S or 50S subunits in the ribosome signpost drug resistance (Lin et al. 2018 ). Although the adaptations in Gram-positive bacteria favor resistance, Gram-negative bacteria continue to remain resistant, owed toward the production of β-lactamases (Maddiboyina et al. 2021a ). Gram-positive bacteria can be inhibited by glycopeptides like Vancomycin and teicoplanin, which use d -alanyl- d -alanine residues to prevent the construction of cell walls. However, glycopeptides don’t crosslink with d -alanylalanine, resulting in later resistance to them. Mutations in DNA gyrase and topoisomerase IV point to resistance to FQ. Due to mutations in genes gyr A and par C (DNA gyrase and topoisomerase IV), there is a replication standstill (Li et al. 2022 ). Tetracycline resistance is revealed by ribosomal protection contraption. There are several RNA polymerase mutations responsible for rifampicin resistance.
Inactivation of enzymes
Antibiotics are deactivated by three major enzymes: Chloramphenicol acetyltransferases (AACs), β-lactamases, and aminoglycoside-modifying enzymes.
Beta-lactamases
β-lactamases hydrolyze almost entire β-lactams, such as penicillins, cephalosporins, monobactams, and carbapenems, with an obligate ester and an amide bond.
Aminoglycoside-modifying enzymes (AGEs): AMEs offer broad-spectrum resistance to AGs and FQs as well as the ability to minimize molecular similarity and binding to the 30S ribosomal subunit (Lee et al. 2018 ).
Chloramphenicol-acetyl-transferases
The enzyme chloramphenicol transacetylase acetylates chloramphenicol hydroxyl groups. Even with adaptations, it remains still inept at connecting with a ribosomal 50S subunit in a proper way (Ghafoori et al. 2021 ).
MRSA antibiotic resistance and antibiotic action mechanisms
MRSA has remained a foremost global healthcare issue for years (Samia et al. 2022 ; Maddiboyina et al. 2021b ). Vancomycin has remained the medication of choice in treating MRSA infection conditions. In clinical management, S. aureus is a multidrug-resistant condition known as a potentially life-threatening superbug (Ahmad-Mansour et al. 2021 ; Maddiboyina et al. 2021c ). The Centers for Disease Control and Prevention (CDC) estimates that more than 75,000 persons in the USA are infected with MRSA every year, with the vast majority of healthcare-associated settings occurring in the geriatric population. The 5-generation cephalosporins and a broad spectrum of antibiotics are beneficial in the management of bacterial infection and MRSA.
Usually, β-lactam antibiotics remain effective against action by S. aureus ; however, other antibiotic agents are also prime for clinical management of staphylococcus infections. These β-lactam antibiotics can bind the intrinsic PBP2a enzyme, the penicillin-binding protein determined through the MecA gene resistance (Fergestad et al. 2020 ; Jones et al. 2010 ). In MRSA infection conditions, Staphylococci express the PCI β- lactamase enzyme adept at hydrolyzing the β-lactam ring, resulting in reduced antibiotic activity and the attainment of an encoding gene-modified penicillin-binding protein (PBP), also identified as PBP2a, as shown in Fig. 4 . PBP2a is still innately resistant to β-lactam ring reticence (Fuda et al. 2005 ). PBP2a detritus plays a part in the growth of β-lactam antibiotics because it neutralizes the PBP enzymes, giving β-lactam antibiotics time to establish cell wall synthesis. It also allows the bacteria to flourish despite the presence of β-lactam inhibitors.
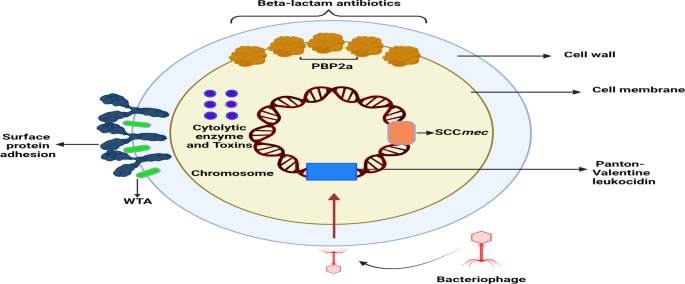
Mechanism through which methicillin resistance in S. aureus evolves
MRSA bacterial infection responds quickly to a modern class of antibiotics and develops a resistance mechanism. MRSA is resistant to primarily endogenous compounds, such as the MecA gene, a novel penicillin-binding protein, and PBP2a. PBP2a endogenous compound, on the other hand, has a low affinity for methicillin conditions; it also allows bacterial cell wall advancement in the presence of antibiotics. Sometimes methicillin resistance improves phenotypes, and PBP2a enzyme production influences antibiotic enzymatic inactivation via inhibition of penicillinase output (Corey et al. 2010 ). This results in penicillin resistance and resistance toward further anti-staphylococcus agents aforementioned as amoxicillin, ampicillin, penicillin V, and aminoglycosides such as gentamicin and amikacin. We used linezolid, daptomycin, and ceftaroline antibiotics as alternative clinical management therapy in severe MRSA infections such as bacteremia, spinal discitis, and MRSA pneumonia (File et al. 2010 ).
Various advancements in the treatment strategies for MRSA
Self-assembling lipid bilayers, or liposomes, can deliver large amounts of antimicrobial drugs to the interior of bacteria by fusion with bacterial cell membranes. Rani et al. provided an overview of prospective liposome targeting strategies for MRSA in their study (Mat Rani et al. 2021 ). Phospholipid nanoparticles synthesized from penicillin G improved cellular uptake of the antibiotic compared to free antibiotic and killed intracellular MRSA in infected A549 lung epithelial cells (Zhang et al. 2019 ). Increased accumulation in thigh tissues infected with MRSA and decreased accumulation in the kidney were observed after administering folic acid-coated lipid NPs containing vancomycin (VAN) (Vanamala et al. 2021 ). These results show that this formulation can overcome the limitations of bacterial resistance and adverse side effects in kidneys caused by the free drug.
Solid lipid nanoparticles (SLNPs)
Lipid and polymeric components constitute SLNPs, which can significantly improve medicine administration and bioavailability. Vancomycin-loaded SLNPs with a mean size of 106.9 ± 1.4 nm and a zeta potential of − 16.5 ± 0.95 mV inhibited the growth of MRSA biofilm for 5 days (Ibrahim et al. 2021 ). This was due to the addition of ascorbyl tocopherol succinate, an adjuvant that significantly increased drug release in an acidified environment associated with controls.
Micelle-like structures
Supramolecular hydrogels, supramolecular micelles, and cyclodextrin (CD) inclusion complexes are some of the most common CD-based controlled release approaches (Gadade and Pekamwar 2020 ). Amphiphilic copolymers of poly(ethylene glycol) and poly(-caprolactone) (PCL) connected with CD that has been capped with adamantane light-triggered and stimulus-responsive release of antibiotics and activation of phenylboronic acid-lactamase inhibitors killed MRSA biofilms by creating reactive oxygen species. β-Cyclodextrin-capped phenylboronic acid-tetraphenylethylene conjugates and are coupled with ampicillin (Chen et al. 2021 ).
Chitosan nanocarriers
Wounds that have bacterial biofilms on them take longer to heal and tend to remain open for longer than they should. The antibacterial activity against MRSA was greatly enhanced by a chitosan (CS) film created by Choi et al. that released nitric oxide (NO) (Choi et al. 2020 ). It was three times as effective as the control film and CS film at inhibiting the growth of biofilms, and it reduced bacterial viability by a factor of 10. Wounds infected with MRSA biofilm treated in vivo with NO-releasing CS film showed increased epithelialization, collagen deposition, decreased wound size, and accelerated biofilm dispersal relative to both untreated and CS film-treated MRSA biofilm-infected wounds (Fahimirad et al. 2021 ). Researchers Vijayakumar et al. observed that CS-Ag nanocomposites had a potent bactericidal impact on MRSA in vitro and in vivo, and that CS-AgNPs with diameters between 10 and 50 nm prevented biofilm formation by MRSA and P. aeruginosa at 100 g/ml (Vijayakumar et al. 2020 ). A nanoscale combination of curcumin and CS placed on a hexagonal ZnO with average particle sizes of 48 nm outperformed amoxicillin against MRSA and E. coli (Karthikeyan et al. 2020 ).
Alginate-loaded nanoparticles
Composite nanofibers (38–105 nm) of alginate and oregano essential oil (EO) with 2–3 wt% of oregano EO showed improved antibacterial activity against Listeria monocytogenes , K. pneumoniae , and Salmonella enterica and significantly improved antibacterial activity against MRSA compared to EO without alginate (Lu et al. 2021 ). The antibiotics amikacin and naproxen were encased in a hydrogel created by grafting phenylboronic acid onto the side chain of an alginate polymer. The hydrogel’s ability to react to changes in pH and reactive oxygen species (ROS) allowed it to inhibit bacterial growth and inflammation (Hu et al. 2020 ).
Cellulose nanoparticles
Natural polymer cellulose is both non-toxic and biodegradable. It is functionalizable, and functionalized derivatives are effective in treating wounds (Momin et al. 2021 ). Norrrahim et al. discovered that dialdehyde nanocrystalline cellulose with increasing amounts of aldehyde groups had potent antibacterial action against Gram-positive pathogens in vitro and reduced the number of MRSA germs on the skin of infected mice models (Norrrahim et al. 2021 ). Bacterial cellulose/polyvinyl alcohol hydrogels loaded with ampicillin killed bacteria more effectively than S. aureus and E. coli because they released 30% of the antibiotic’s entire dose over 120 h (Tamahkar 2021 ).
Hyaluronic acid-based nanocarriers
Hyaluronic acid (HA) polymersomes were used to encapsulate vancomycin (Placha and Jampilek 2021 ). These polymersomes were spherical in shape, self-assembled, and had a negative zeta potential. These polymersomes were 72 times more effective in combating MRSA than free medication, had a larger effect on the bacterium’s membrane, and released their contents steadily over the course of three days. Self-assembling conjugated oligo(thiophene ethynylene) (OTE)-covalently modified HA (OTE-HA) NPs have been discovered that they prevent the premature release of the bactericide (Yuan et al. 2021 ).
Silver nanoparticles
Particle sizes of the colloidal Ag produced from the Corymbia maculata aqueous leaf extract were 40 mm and 11–16 nm, respectively, when the colloidal Ag was prepared, and 11–16 nm when the dried Ag was utilized. The MIC and MBEC values against plantonic P. aeruginosa chronic rhinosinusitis clinical isolates ranged from 0.2 to 3, indicating improved antibacterial action (Feizi et al. 2021 ). Similarly, green AgNPs NPs (3–25 nm) derived from extracts of Pyrenacantha grandiflora tuber demonstrated significant antibacterial action against methicillin-resistant Staphylococcus aureus and other MDR bacteria (Murei et al. 2021 ). To kill P. aeruginosa, L. monocytogenes , and MRSA, researchers found that using a 50-mW 400-nm femtosecond laser in conjunction with AgNPs was more effective than using AgNPs alone (El-Gendy et al. 2021 ). Gentamicin-filled gentamicin-virus-shaped mesoporous SiO2-coated Ag nanocubes are more effective than other antibiotics at killing Escherichia coli and methicillin-resistant Staphylococcus aureus (Wang et al. 2021 ). For optimal MRSA killing, hybrids of graphene quantum dots (GRQDs) and silver nanoparticles (AgNPs) were shown to be most effective (Zhong et al. 2020 ).
MRSA transmission and colonization; the role of animals and the environment in resistance transmission
Despite being found in most people and animals, S. aureus is still one of the utmost customary bacterial infections (Chang et al. 2003 ). Around 30% of the population is infected with methicillin-resistant S. aureus , and these bacteria increase healthcare expenditures while making people sick. This case’s transmission involves nasal epithelial cells and components related to the synthesis of proteins and the cell’s surfaces. MRSA colonization is still heavily reliant on nasal carriage, with up to 80% of the human population’s transmissions occurring because of this. Persistent carriers of MRSA remain at greater risk of infection than surgical patients, intensive care unit patients, and patients on dialysis (Wong et al. 2023 ). It is nose-picking patients, however, who recurrently increase the colonized S. aureus infection from one individual to another through direct hand contact, as shown in the study’s results. As well humans, MRSA colonization occurs in animals such as dogs, cats, birds, and cows (Crespo-Piazuelo and Lawlor 2021 ). Direct contact with infected animals or humans has stood reported as a means of spreading MRSA infection. A high rate of MRSA illness in humans has also been found in recent prevalence studies due to close contact with animals (Garoy et al. 2019 ).
Antibiotics resistance as other languages of microbial communication
The clinician has extensively identified that microbial communication-specific bacteria, viruses, and fungi remain distressingly resistant to the antibiotics employed to tackle them. In 2015, a World Health Organization survey in 12 nations emphasized people’s unawareness of the languages of antibiotic resistance (Michaelidou et al. 2020 ). Antibiotic drug resistance is caused by the fact that half of the world’s population has never heard of the languages of antibiotics. A small amount of clear and explicit phrasing words helps protect the overall effort, while antibiotic resistance remains intense in language. Antibiotic resistance is interconnected with the bacterial cell of identical or diverse species through the language used by these bacterial cells. This language is intended to obstruct novel treatments by reducing antibiotic resistance in distinct bacteria and preventing the correspondence of specific resistance amid diverse bacteria. A person’s healing time is shortened when bacterial contagions are considered. A more widespread indication will be more helpful in achieving this goal if the inhibitors can mark a particle specific to a particular bacterial group. So, putrescine and further polyamines seem to remain likely targets for specific inhibitors assumed distinct environments and distinct recently recognized functions in the correspondence of antibiotic resistance. Polymyxin B resistance was reduced in B. cenocepacia when ornithine decarboxylase was pharmacologically inhibited (ornithine is converted into putrescine). There is a greater chance that similar inhibitors, such as putrescine, and further small particles corresponding to antibiotic resistance, will remain reliable for human use as antibiotic auxiliaries due to this study. Investigating the molecular source of antibiotic resistance chemical correspondence will be necessary to explain the intricate chemical indications and their part in the extent of antibiotic resistance amid bacteria prominent toward the failure of antimicrobial remedy (Jhawat et al. 2020 ).
Current MRSA clinical executive recommendations
Despite its ubiquity, treating MRSA is still a severe problem for the global public (Guo et al. 2020 ). Diagnoses were made after a thorough examination of symptoms, and of those diagnosed, the cases of those suffering from the most severe infections were treated first; MRSA treatment has evolved. The Enterococcus, Staph aureus, Klebsiella pneumoniae, and acinetobacter species in question are ESKAPE pathogens, meaning they are complicated to treat. There are various methods for diagnosing MRSA, including clinical symptoms, tissue samples (or) nasal secretions expending the microbial culturing practice, and antibiotic resistance of bacterial infections. MRSA skin infections and nasal colonization conditions were responsible for 60% of the population’s diagnosis. There are two types of antibiotics available to treat MRSA (Choo and Chambers 2016 ).
An older antibiotic agent for MRSA treatment
Some outpatients continue to receive oral antibiotics for monotherapy, such as clindamycin, trimethoprim-sulfamethoxazole, doxycycline, and aminocycline, despite these antibiotics’ ineffectiveness. For systemic MRSA, the majority of clinicians commonly prescribed vancomycin antibiotics. The Infectious Disease Society of America also endorses Vancomycin (2 μg/ml) as the first medication to cure MRSA. In numerous global surveillance studies, vancomycin dosing (to a maximum of 15 µg per millilitre per day) was also beneficial in treating MRSA (Hindy et al. 2022 ).
Newer antibiotics for the treatment of MRSA
S. aureus is still a Gram-positive bacterium notorious for its unusually severe pathogenic constitution (Yuan et al. 2021 ). MRSA remains liable for various ailments, including skin infections and the development of multiple organ diseases; it remains the second prominent source of illness and death worldwide, and healthcare professionals face complex challenges in managing MRSA infections in multiple drug-resistant conditions, which can be fatal (Lee et al. 2018 ). As a result, there is still a significant need to advance novel and expedient drug management therapy for MRSA infection. A new promising antibacterial therapeutic management system was introduced through the FDA to treat MRSA infection conditions, and the therapy management system is also used in different microbial resistance conditions (Maddiboyina et al. 2022b ).
The FDA has permitted five anti-infective agents for treating MRSA-related multidrug resistance: linezolid, daptomycin, tigecycline, telavancin, and ceftaroline. Linezolid, one of the new antibiotic agents available in intravenous formulation, is still available in oral and intravenous preparations. This antibiotic could be used as a first-line treatment for beta-hemolytic streptococci and MRSA. However, it has been clinically prescribed to be selective in treating acute MRSA, and these antibiotic agents are used as a secondary choice based on the patient’s financial budget (Wunderink et al. 2012 ). Table 1 summarizes the therapeutic management outcomes of these agents when compared to vancomycin. Many new antibiotic agents have remained familiarized in the market to tackle MRSA, and all of them represent promising options for improving the antibiotic mechanism of action.
Nanotechnology in MRSA theranostic applications
Nanoparticle-based antibiotic formulation paradigms have unavoidably adopted a prevalent extent of exploration in clinical biomedical disciplines. Clinical research has primarily focused on nanochemistry to control infectious diseases (or) multiple drug resistance bacterial infection conditions and cancer therapy. The primary application of nanoscience and nanotechnology drug delivery systems is controlling contagious diseases (Tong et al. 2015 ). One of the major clinical problems is S. aureus associated with MRSA infection, so the determining prerequisite for novel approach therapy for efficiently allocating through MRSA infection is emphasized. They are working on developing a nanoparticle antibiotic drug delivery system. The unique size and properties of nanobiotic drugs improve antibacterial activity, increase the antibacterial drug agonist mechanism of action, and decrease systemic toxicity (Mosselhy et al. 2021 ). The nanoparticles also increase the bioavailability of the drug in the serum.
MRSA detection using nanomaterial’s
This includes photodynamic therapy, light-oxygen therapies, and reactive oxygen species (ROS). Nanomaterials significantly increase accuracy and precision in diagnostic colorimetric assays and PCR markers. Advances in nanomaterials have facilitated the development of biosensor devices characterized by excellent optical, electrochemical, and mechanical properties. Several electrochemical, fluorescence, and mechanical sensors are still developed to detect MRSA biomarkers. Numerous advanced practices must remain engaged in identifying and exemplifying exceptional in a clinical drawing, MRSA (Gill et al. 2019 ).
Excellent results are achieved using colorimetric nanomaterial-based MRSA sensors and outcomes without the need for complex equipment. Despite rapid advancements in nanomaterial-based biosensor mechanisms, no suitable MRSA sensor has been found since overlooked and has yet to be addressed. One issue is that several advanced biosensors require intensifying genes or DNA before they can be recognized. Nanomaterial-based biosensors should retain several essential characteristics, including time, selectivity, and reduced sensitivity.
Nanomaterial-based MRSA treatment therapy
New therapeutic management causes irreversible damage to bacteria’s bimolecular synthesis processes, such as DNA, mRNA, and lipid synthesis. The US Food and Drug Administration also approved nanoparticulate system therapy for MRSA infection. A novel nanoparticle biodegradable polymer-based drug delivery system can improve permeation, 100% bioavailability, good controlled release, and improved clinical outcomes index. This nanotechnology treatment also has lower therapeutic toxicity levels when compared to crude drugs. Only a few drugs are available in a nanoform, such as Vancomycin, gold therapy, amoxicillin, and chloramphenicol. The drugs have specific targeting properties and a completely bioavailable compound. Most hospital clinical management of MRSA life-threatening conditions employs nanotechnology formulation therapies (Singh et al. 2021 ).
Despite the commitment of several years, work has centered on improving the treatment of MRSA with a focus on increased activity and efficacy. Nano-technology, which the FDA permitted to treat MRSA and S. aureus infection in 1994, has now been implemented in other therapeutic applications. The increased effects result from their size, structure, and protective inner environment, and the nanosubstance improves drug action in cell sites, organs, and tissues.
The nanoparticles also improve serum solubility, which prolongs the drug’s therapeutic effects and is a sustained, controlled-release dosage form, capsule-based delivery formulation. This means that nanoparticles can remain employed in the therapy of intracellular microbial ailments. In 1994, vancomycin and teicoplanin antibiotics were reported in a nanoparticle formulation to treat MRSA infections (Onyeji et al. 1994 ). The medication remains multilamellar liposome, which has improved beside MRSA bacteria positioned sites contained by human macrophages, whereas liposomes enclosing vancomycin (200 and 800 g/ml concentration) drug reduced MRSA survival.
Esmaeili et al. used poly- d , l -lactic-co-glycolic acid (PLGA) nanoparticles to deliver rifampicin. Rifampicin remains employed to cure mycobacterium ailments and leprosy, and fusidic acid remains to medicate MRSA ailments (Esmaeili et al. 2007 ). In vitro antibacterial activity, an identical explore by Duran et al. in 2008 revealed that such rifampicin assimilated into rifampicin nanoparticles (20–60 m) formulation maintains anti-MRSA bioactivity while decreasing cytotoxicity of rifampicin(Durán et al. 2008 ).
Clinical aspects and recent advancements of MRSA
MRSA infection has increased medication resistance over the last few decades, and only a few antimicrobial drugs are available to treat MRSA infections. Anti-staphylococcus drugs should be used more widely in the therapy of MRSA ailments. Several clinical trials have continued to develop new-generation antibiotics to combat MSRA.
The researchers are continuing to develop a currently available antibiotics arsenal to be effective drug resistance bacterial infections, in here drug discovery processes considered the four essential points, mainly (i) reformulation of infections diseased remedy, (ii) curtailment of the curative supervisions, (iii) reduced the drug side effects and increased the drug effectiveness parameters, and (iv) minimizing the practice of drugs. The ultimate goal of a clinical trial is to find (or develop) a new antibacterial agent to treat MRSA infection. Table 2 contains the most recent clinical trials on anti-staphylococcal drugs and the infection against bacterial growth inhibitory activities drugs against gram-positive bacteria, including MRSA infections (Kurosu et al. 2013 ).
The cell wall glycopolymer wall teichoic acid (WTA) has been identified as a dominant surface antigen, and immunoproteomics studies have assisted in elucidating the most immunogenic and protective S. aureus antigens. In addition to vaccinations, bacteriophages or lytic proteins produced from bacteriophages may be employed for novel preventive measures, such as nasal MRSA decontamination in a time of rising mupirocin resistance (Ahmed 2022 ). Bacterial two-component systems play a central role in the adaptative response of bacteria to their ever-changing environment. The maleimide-based compounds are evaluated against a model histidine kinase, HK853, in vitro and in silico. The most potent leads were then assessed for their ability to decrease the pathogenicity and virulence of MRSA (Espinasse et al. 2023 ). Aaron et al. developed the purine-derived signaling molecules c-di-AMP and (p)ppGpp control mecA/PBP2a-mediated β-lactam resistance in MRSA raise the possibility that purine availability can control antibiotic susceptibility(Nolan et al. 2023 ). Fan et al. found that uracil could synergize with aminoglycosides to kill MRSA (USA300) by 400-fold. Reprogramming metabolomics displayed uracil reprogrammed bacterial metabolism, especially enhanced the tricarboxylic acid (TCA) cycle to elevate NADH production and proton motive force, thereby promoting the uptake of antibiotics. Furthermore, uracil increased cellular respiration and ATP production, resulting the generation of reactive oxygen species (ROS). Thus, the combined activity of uracil and antibiotics induced bacterial death. Inhibition of the TCA cycle or ROS production could attenuate bactericidal efficiency. Moreover, uracil exhibited bactericidal activity in cooperation with aminoglycosides against other pathogenic bacteria (Fan et al. 2023 ).
Conclusions
MRSA remnants among multidrug-resistant entities unquestionably necessitate increased efforts for discovering and advancing novel antibiotics and advanced anticipatory practices. MRSA is still considered a foremost risk to public health because of its extreme adaptability and ability to progress resistance. It has designed its public home and established an innovative environmental niche in animals. As a result, it remains imperative for prevailing MRSA exploration to describe aspects outlining the virulence of the intact type of contagious MRSA strains. Substitute therapeutic procedures for MRSA are still developing, but there is no timetable or guarantee that they will be effective in a clinical trial. Clinicians and researchers are constantly reminded that preventing MRSA infections is preferable to treating them. A temporary state of well-being may be provided by the current therapeutic array and the total number of medications in advance, but this assumption could lead to the demise of the healthcare organization. MRSA will be a critical area of investigation and advancement for the foreseeable future to stay ahead of the problem.
Auxiliary research is still required to constantly revise MRSA’s ability to source contagion and antibiotic resistance conduits and maintain the progress of innovative drugs other than MRSA contagion. The advancement of innovative medicines has given doctors more options for dealing with MRSA infections, providing superior fortification for human health. However, the efficacy and safety of medications necessitate further clinical investigation.
Availability of data and materials
Data available on request from the authors.
Abbreviations
Methicillin-resistant Staphylococcus aureus
Aminoglycosides
Fluoroquinolone
Aminoglycoside-modifying enzymes
Centers for Disease Control and Prevention
Penicillin-binding protein
Solid lipid nanoparticles
Cyclodextrin
Poly(-caprolactone)
Nitric oxide
Reactive oxygen species
Hyaluronic acid
Oligo(thiophene ethynylene)
Poly-lactic-co-glycolic acid
Ahmed MM (2022) MRSA infections: priorities and future approaches for research. Gastroenterol Hepatol Open Access. https://doi.org/10.15406/ghoa.2022.13.00523
Article Google Scholar
Ahmad-Mansour N, Loubet P, Pouget C, Dunyach-Remy C, Sotto A, Lavigne J-P, Molle V (2021) Staphylococcus aureus toxins: an update on their pathogenic properties and potential treatments. Toxins. https://doi.org/10.3390/toxins13100677
Article PubMed PubMed Central Google Scholar
Blaskovich MAT, Hansford KA, Butler MS, Jia Z, Mark AE, Cooper MA (2018) Developments in glycopeptide antibiotics. ACS Infect Dis 4:715–735
Article CAS PubMed PubMed Central Google Scholar
Bush NG, Diez-Santos I, Abbott LR, Maxwell A (2020) Quinolones: mechanism, lethality and their contributions to antibiotic resistance. Molecules. https://doi.org/10.3390/molecules25235662
Chang S, Sievert DM, Hageman JC et al (2003) Infection with vancomycin-resistant Staphylococcus aureus containing the vanA resistance gene. N Engl J Med. https://doi.org/10.1056/nejmoa025025
Article PubMed Google Scholar
Chen M, Qiu B, Zhang Z, Xie S, Liu Y, Xia T, Li X (2021) Light-triggerable and pH/lipase-responsive release of antibiotics and β-lactamase inhibitors from host-guest self-assembled micelles to combat biofilms and resistant bacteria. Chem Eng J. https://doi.org/10.1016/j.cej.2021.130330
Choi M, Hasan N, Cao J, Lee J, Hlaing SP, Yoo JW (2020) Chitosan-based nitric oxide-releasing dressing for anti-biofilm and in vivo healing activities in MRSA biofilm-infected wounds. Int J Biol Macromol. https://doi.org/10.1016/j.ijbiomac.2019.10.009
Choo EJ, Chambers HF (2016) Treatment of methicillin-resistant Staphylococcus aureus bacteremia. Infect Chemother 48:267–273
Cong Y, Yang S, Rao X (2020) Vancomycin resistant Staphylococcus aureus infections: a review of case updating and clinical features. J Adv Res 21:169–176
Corey GR, Wilcox M, Talbot GH, Friedland HD, Baculik T, Witherell GW, Critchley I, Das AF, Thye D (2010) Integrated analysis of CANVAS 1 and 2: Phase 3, multicenter, randomized, double-blind studies to evaluate the safety and efficacy of ceftaroline versus vancomycin plus aztreonam in complicated skin and skin-structure infection. Clin Infect Dis. https://doi.org/10.1086/655827
Cowan ST, Shaw C, Williams RE (1954) Type strain for Staphylococcus aureus rosenbach. J Gen Microbiol. https://doi.org/10.1099/00221287-10-1-174
Crespo-Piazuelo D, Lawlor PG (2021) Livestock-associated methicillin-resistant Staphylococcus aureus (LA-MRSA) prevalence in humans in close contact with animals and measures to reduce on-farm colonisation. Ir Vet J 74:21
Durán N, Alvarenga MA, Da Silva EC, Melo PS, Marcato PD (2008) Microencapsulation of antibiotic rifampicin in poly(3-hydroxybutyrate-co-3-hydroxyvalerate). Arch Pharm Res. https://doi.org/10.1007/s12272-001-2137-7
El-Gendy AO, Samir A, Ahmed E, Enwemeka CS, Mohamed T (2021) The antimicrobial effect of 400 nm femtosecond laser and silver nanoparticles on gram-positive and gram-negative bacteria. J Photochem Photobiol B Biol. https://doi.org/10.1016/j.jphotobiol.2021.112300
Esmaeili F, Atyabi F, Dinarvand R (2007) Preparation of PLGA nanoparticles using TPGS in the spontaneous emulsification solvent diffusion method. J Exp Nanosci. https://doi.org/10.1080/17458080701393137
Espinasse A, Goswami M, Yang J, Vorasin O, Ji Y, Carlson EE (2023) Targeting multidrug resistant Staphylococcus infections with bacterial histidine kinase inhibitors. Chem Sci. https://doi.org/10.1039/D2SC05369A
Fahimirad S, Abtahi H, Satei P, Ghaznavi-Rad E, Moslehi M, Ganji A (2021) Wound healing performance of PCL/chitosan based electrospun nanofiber electrosprayed with curcumin loaded chitosan nanoparticles. Carbohydr Polym. https://doi.org/10.1016/j.carbpol.2021.117640
Fan L, Pan Z, Liao X, Zhong Y, Guo J, Pang R, Chen X, Ye G, Su Y (2023) Uracil restores susceptibility of methicillin-resistant Staphylococcus aureus to aminoglycosides through metabolic reprogramming. Front Pharmacol. https://doi.org/10.3389/fphar.2023.1133685
Feizi S, Cooksley CM, Bouras GS, Prestidge CA, Coenye T, Psaltis AJ, Wormald PJ, Vreugde S (2021) Colloidal silver combating pathogenic Pseudomonas aeruginosa and MRSA in chronic rhinosinusitis. Colloids Surfaces B Biointerfaces. https://doi.org/10.1016/j.colsurfb.2021.111675
Fergestad ME, Stamsås GA, Morales Angeles D, Salehian Z, Wasteson Y, Kjos M (2020) Penicillin-binding protein PBP2a provides variable levels of protection toward different β-lactams in Staphylococcus aureus RN4220. Microbiologyopen 9:e1057
File TM, Low DE, Eckburg PB, Talbot GH, Friedland HD, Lee J, Llorens L, Critchley I, Thye D (2010) Integrated analysis of FOCUS 1 and FOCUS 2: Randomized, doubled-blinded, multicenter phase 3 trials of the efficacy and safety of ceftaroline fosamil versus ceftriaxone in patients with community-acquired pneumonia. Clin Infect Dis. https://doi.org/10.1086/657313
Fuda CCS, Fisher JF, Mobashery S (2005) β-Lactam resistance in Staphylococcus aureus : the adaptive resistance of a plastic genome. Cell Mol Life Sci. https://doi.org/10.1007/s00018-005-5148-6
Gadade DD, Pekamwar SS (2020) Cyclodextrin based nanoparticles for drug delivery and theranostics. Adv Pharm Bull. https://doi.org/10.34172/apb.2020.022
Garoy EY, Gebreab YB, Achila OO, Tekeste DG, Kesete R, Ghirmay R, Kiflay R, Tesfu T (2019) Methicillin-resistant Staphylococcus aureus (MRSA): prevalence and antimicrobial sensitivity pattern among patients-a multicenter study in Asmara, Eritrea. Can J Infect Dis Med Microbiol 2019:8321834
Ghafoori SM, Robles AM, Arada AM et al (2021) Structural characterization of a Type B chloramphenicol acetyltransferase from the emerging pathogen Elizabethkingia anophelis NUHP1. Sci Rep 11:9453
Article ADS CAS PubMed PubMed Central Google Scholar
Gill AAS, Singh S, Thapliyal N, Karpoormath R (2019) Nanomaterial-based optical and electrochemical techniques for detection of methicillin-resistant Staphylococcus aureus : a review. Microchim Acta. https://doi.org/10.1007/s00604-018-3186-7
Guo Y, Song G, Sun M, Wang J, Wang Y (2020) Prevalence and therapies of antibiotic-resistance in Staphylococcus aureus . Front Cell Infect Microbiol 10:107. https://doi.org/10.3389/fcimb.2020.00107
Hindy JR, Haddad SF, Kanj SS (2022) New drugs for methicillin-resistant Staphylococcus aureus skin and soft tissue infections. Curr Opin Infect Dis 35:112–119. https://doi.org/10.1097/QCO.0000000000000800
Article CAS PubMed Google Scholar
Hu C, Zhang F, Long L, Kong Q, Luo R, Wang Y (2020) Dual-responsive injectable hydrogels encapsulating drug-loaded micelles for on-demand antimicrobial activity and accelerated wound healing. J Control Release. https://doi.org/10.1016/j.jconrel.2020.05.010
Ibrahim UH, Devnarain N, Omolo CA, Mocktar C, Govender T (2021) Biomimetic pH/lipase dual responsive vitamin-based solid lipid nanoparticles for on-demand delivery of vancomycin. Int J Pharm. https://doi.org/10.1016/j.ijpharm.2021.120960
Iyer RN (2022) 7.02 - Beta lactam. Kenakin TBT-CP. Elsevier, Oxford, pp 3–63
Google Scholar
Jhawat V, Gulia M, Gupta S, Maddiboyina B, Dutt R (2020) Integration of pharmacogenomics and theranostics with nanotechnology as quality by design (QbD) approach for formulation development of novel dosage forms for effective drug therapy. J Control Release. https://doi.org/10.1016/j.jconrel.2020.08.039
Jones RN, Mendes RE, Sader HS (2010) Ceftaroline activity against pathogens associated with complicated skin and skin structure infections: results from an international surveillance study. J Antimicrob Chemother. https://doi.org/10.1093/jac/dkq252
Karthikeyan C, Varaprasad K, Akbari-Fakhrabadi A, Hameed ASH, Sadiku R (2020) Biomolecule chitosan, curcumin and ZnO-based antibacterial nanomaterial, via a one-pot process. Carbohydr Polym. https://doi.org/10.1016/j.carbpol.2020.116825
Kurosu M, Siricilla S, Mitachi K (2013) Advances in MRSA drug discovery: Where are we and where do we need to be? Expert Opin Drug Discov. https://doi.org/10.1517/17460441.2013.807246
Lee AS, de Lencastre H, Garau J, Kluytmans J, Malhotra-Kumar S, Peschel A, Harbarth S (2018) Methicillin-resistant Staphylococcus aureus . Nat Rev Dis Prim 4:18033
Li J, Wei Y, Wang J, Li Y, Shao G, Feng Z, Xiong Q (2022) Characterization of mutations in DNA gyrase and topoisomerase IV in field strains and in vitro selected quinolone-resistant mycoplasma hyorhinis mutants. Antibiotics. https://doi.org/10.3390/antibiotics11040494
Lin J, Zhou D, Steitz TA, Polikanov YS, Gagnon MG (2018) Ribosome-targeting antibiotics: modes of action, mechanisms of resistance, and implications for drug design. Annu Rev Biochem 87:451–478
Lu H, Butler JA, Britten NS, Venkatraman PD, Rahatekar SS (2021) Natural antimicrobial nano composite fibres manufactured from a combination of alginate and oregano essential oil. Nanomaterials. https://doi.org/10.3390/nano11082062
Maddiboyina B, Asthana A, Asthana GS, Singh S, Ramya M, Sunnapu O, Kotla N (2015) Formulation and characterization of polycarbophil coated mucoadhesive microspheres of repaglinide. J Pharm Sci Res 7(11):972–977
CAS Google Scholar
Maddiboyina B, Hanumanaik M, Nakkala RK et al (2020) Formulation and evaluation of gastro-retentive floating bilayer tablet for the treatment of hypertension. Heliyon 6:e05459
Maddiboyina B, Shanmugapriya J, Rasala S, Sivaraman G (2021a) Bioinspired Nanomaterials for Drug Delivery. In: Bioinspired Nanomater. Synth. Emerg. Appl., Materials. Materials Research Forum LLC, pp 63–95
Maddiboyina B, Desu PK, Vasam M, Challa VT, Surendra AV, Rao RS, Alagarsamy S, Jhawat V (2021b) An insight of nanogels as novel drug delivery system with potential hybrid nanogel applications. J Biomater Sci Polym Ed. https://doi.org/10.1080/09205063.2021.1982643
Maddiboyina B, Jhawat V, Nakkala RK, Desu PK, Gandhi S (2021c) Design expert assisted formulation, characterization and optimization of microemulsion based solid lipid nanoparticles of repaglinide. Prog Biomater. https://doi.org/10.1007/s40204-021-00174-3
Maddiboyina B, Nakkala RK, Sivaraman G (2022a) Biomass-derived mesoporous carbon nanomaterials for drug delivery and imaging applications. In: Biomass‐derived carbon mater. pp 129–146
Maddiboyina B, Sunaapu OP, Chandrashekharappa S, Sivaraman G (2022b) Advantages and limitations of functionalized nanomaterials based electrochemical sensors environmental monitoring. In: Functionalized nanomaterial-based electrochemical sensors, Princ Fabr Methods, Appl. https://doi.org/10.1016/B978-0-12-823788-5.00016-8
Maddiboyina B, Vanamamalai HK, Roy H, Ramaiah GS, Kavisri M, Moovendhan M (2023) Food and drug industry applications of microalgae spirulina platensis: a review. J Basic Microbiol. https://doi.org/10.1002/jobm.202200704
Maddina BY, Asthana GS, Asthana A (2016) A Review on current scenario of spirulina drug delivery systems. World J Pharm Sci 4:86–89
Mat Rani NNI, Mustafa Hussein Z, Mustapa F, Azhari H, Sekar M, Chen XY, Mohd Amin MCI (2021) Exploring the possible targeting strategies of liposomes against methicillin-resistant Staphylococcus aureus (MRSA). Eur J Pharm Biopharm. https://doi.org/10.1016/j.ejpb.2021.04.021
Maya-Martinez R, Alexander JAN, Otten CF et al (2019) Recognition of peptidoglycan fragments by the transpeptidase PBP4 From Staphylococcus aureus . Front Microbiol 9:3223. https://doi.org/10.3389/fmicb.2018.03223
Michaelidou M, Karageorgos SA, Tsioutis C (2020) Antibiotic use and antibiotic resistance: public awareness survey in the Republic of Cyprus. Antibiotics. https://doi.org/10.3390/antibiotics9110759
Momin M, Mishra V, Gharat S, Omri A (2021) Recent advancements in cellulose-based biomaterials for management of infected wounds. Expert Opin Drug Deliv. https://doi.org/10.1080/17425247.2021.1989407
Mosselhy DA, Assad M, Sironen T, Elbahri M (2021) Nanotheranostics: a possible solution for drug-resistant Staphylococcus aureus and their Biofilms? Nanomater. https://doi.org/10.3390/nano11010082
Murei A, Pillay K, Govender P, Thovhogi N, Gitari WM, Samie A (2021) Synthesis, characterization and in vitro antibacterial evaluation of Pyrenacantha grandiflora conjugated silver nanoparticles. Nanomaterials. https://doi.org/10.3390/nano11061568
Nolan AC, Zeden MS, Kviatkovski I, Campbell C, Urwin L, Corrigan RM, Gründling A, O’Gara JP (2023) Purine nucleosides interfere with c-di-AMP levels and act as adjuvants to re-sensitize MRSA To β-lactam antibiotics. Mbio. https://doi.org/10.1128/mbio.02478-22
Norrrahim MNF, Nurazzi NM, Jenol MA, Farid MAA, Janudin N, Ujang FA, Yasim-Anuar TAT, Syed Najmuddin SUF, Ilyas RA (2021) Emerging development of nanocellulose as an antimicrobial material: an overview. Mater Adv. https://doi.org/10.1039/d1ma00116g
Ogston A (1882) Micrococcus Poisoning. J Anat Physiol 17(Pt 1):24–58
CAS PubMed PubMed Central Google Scholar
Onyeji CO, Nightingale CH, Marangos MN (1994) Enhanced killing of methicillin-resistant Staphylococcus aureus in human macrophages by liposome-entrapped vancomycin and teicoplanin. Infection. https://doi.org/10.1007/BF01715542
Ovung A, Bhattacharyya J (2021) Sulfonamide drugs: structure, antibacterial property, toxicity, and biophysical interactions. Biophys Rev 13:259–272
Placha D, Jampilek J (2021) Chronic inflammatory diseases, anti-inflammatory agents and their delivery nanosystems. Pharmaceutics. https://doi.org/10.3390/pharmaceutics13010064
Raineri EJM, Altulea D, van Dijl JM (2022) Staphylococcal trafficking and infection-from “nose to gut” and back. FEMS Microbiol Rev. https://doi.org/10.1093/femsre/fuab041
Report NDS (2020) Centers for Disease Control and Prevention. National Diabetes Statistics Report, 2020. Atlanta, GA: Centers for Disease Control and Prevention, U.S. Dept of Health and Human Services; 2020. Natl. Diabetes Stat. Rep.
Reygaert WC (2018) An overview of the antimicrobial resistance mechanisms of bacteria. AIMS Microbiol 4:482–501
Roger C, Roberts JA, Muller L (2018) Clinical pharmacokinetics and pharmacodynamics of oxazolidinones. Clin Pharmacokinet 57:559–575
Roy H, Nayak BS, Maddiboyina B, Nandi S (2022) Chitosan based urapidil microparticle development in approach to improve mechanical strength by cold hyperosmotic dextrose solution technique. J Drug Deliv Sci Technol 76:103745
Article CAS Google Scholar
Sakr A, Brégeon F, Mège JL, Rolain JM, Blin O (2018) Staphylococcus aureus nasal colonization: an update on mechanisms, epidemiology, risk factors, and subsequent infections. Front Microbiol 9:2419. https://doi.org/10.3389/fmicb.2018.02419
Samia NI, Robicsek A, Heesterbeek H, Peterson LR (2022) Methicillin-resistant staphylococcus aureus nosocomial infection has a distinct epidemiological position and acts as a marker for overall hospital-acquired infection trends. Sci Rep 12:17007
Sanga B, Kharel MKBT-RM in BS (2022) Chloramphenicol. https://doi.org/10.1016/B978-0-12-824315-2.00349-3
Singh S, Numan A, Somaily HH, Gorain B, Ranjan S, Rilla K, Siddique HR, Kesharwani P (2021) Nano-enabled strategies to combat methicillin-resistant Staphylococcus aureus . Mater Sci Eng C 129:112384
Tamahkar E (2021) Bacterial cellulose/poly vinyl alcohol based wound dressings with sustained antibiotic delivery. Chem Pap. https://doi.org/10.1007/s11696-021-01631-w
Tong SYC, Davis JS, Eichenberger E, Holland TL, Fowler VG (2015) Staphylococcus aureus infections: epidemiology, pathophysiology, clinical manifestations, and management. Clin Microbiol Rev. https://doi.org/10.1128/CMR.00134-14
Turner NA, Sharma-Kuinkel BK, Maskarinec SA, Eichenberger EM, Shah PP, Carugati M, Holland TL, Fowler VG (2019) Methicillin-resistant Staphylococcus aureus : an overview of basic and clinical research. Nat Rev Microbiol 17:203–218
Vanamala K, Bhise K, Sanchez H, Kebriaei R, Luong D, Sau S, Abdelhady H, Rybak MJ, Andes D, Iyer AK (2021) Folate functionalized lipid nanoparticles for targeted therapy of methicillin-resistant staphylococcus aureus . Pharmaceutics. https://doi.org/10.3390/pharmaceutics13111791
Vijayakumar S, Malaikozhundan B, Parthasarathy A, Saravanakumar K, Wang MH, Vaseeharan B (2020) Nano biomedical potential of biopolymer chitosan-capped silver nanoparticles with special reference to antibacterial, antibiofilm, anticoagulant and wound dressing material. J Clust Sci. https://doi.org/10.1007/s10876-019-01649-x
Wan TW, Teng LJ, Yamamoto T (2021) Unique surface structures of community-associated methicillin-resistant Staphylococcus aureus ST8/SCCmecIVl. J Microbiol Immunol Infect. https://doi.org/10.1016/j.jmii.2020.09.008
Wang P, Jiang S, Li Y, Luo Q, Lin J, Hu L, Liu X, Xue F (2021) Virus-like mesoporous silica-coated plasmonic Ag nanocube with strong bacteria adhesion for diabetic wound ulcer healing. Nanomed Nanotechnol Biol Med. https://doi.org/10.1016/j.nano.2021.102381
Williams VR, Callery S, Vearncombe M, Simor AE (2009) The role of colonization pressure in nosocomial transmission of methicillin-resistant Staphylococcus aureus . Am J Infect Control. https://doi.org/10.1016/j.ajic.2008.05.007
Wong YT, Yeung CS, Chak WL, Cheung CY (2023) Methicillin-resistant Staphylococcus aureus nasal carriage among patients on haemodialysis with newly inserted central venous catheters. Int Urol Nephrol. https://doi.org/10.1007/s11255-023-03521-4
Wunderink RG, Niederman MS, Kollef MH, Shorr AF, Kunkel MJ, Baruch A, McGee WT, Reisman A, Chastre J (2012) Linezolid in methicillin-resistant staphylococcus aureus nosocomial pneumonia: a randomized, controlled study. Clin Infect Dis. https://doi.org/10.1093/cid/cir895
Yuan Q, Zhao Y, Zhang Z, Tang Y (2021) On-demand antimicrobial agent release from functionalized conjugated oligomer-hyaluronic acid nanoparticles for tackling antimicrobial resistance. ACS Appl Mater Interfaces. https://doi.org/10.1021/acsami.0c19283
Zhang C, Zhao W, Bian C, Hou X, Deng B, McComb DW, Chen X, Dong Y (2019) Antibiotic-derived lipid nanoparticles to treat intracellular Staphylococcus aureus . ACS Appl Bio Mater. https://doi.org/10.1021/acsabm.8b00821
Zhong X, Tong C, Liu T, Li L, Liu X, Yang Y, Liu R, Liu B (2020) Silver nanoparticles coated by green graphene quantum dots for accelerating the healing of: MRSA-infected wounds. Biomater Sci. https://doi.org/10.1039/d0bm01398f
Zimmermann P, Ziesenitz VC, Curtis N, Ritz N (2018) The Immunomodulatory effects of macrolides—a systematic review of the underlying mechanisms. Front Immunol 9:302. https://doi.org/10.3389/fimmu.2018.00302
Download references
Acknowledgements
The authors thank Freyr Solutions for providing the opportunity and resources; Nirmala College of Pharmacy for support. The figures were created with the support of BioRender.com.
No funds, grants, or other support was received.
Author information
Balaji Maddiboyina, Harekrishna Roy, Ramaiah have contributed equally to this work.
Authors and Affiliations
Freyr Global Regulatory Solutions & Services, Phoenix SEZ, HITEC City 2, Gachibowli, Hyderabad, Telangana, 500081, India
Balaji Maddiboyina, M. Ramaiah, C. N. Sarvesh & Sahasra Hanuman Kosuru
Nirmala College of Pharmacy, Mangalagiri, Guntur, Andhra Pradesh, India
Harekrishna Roy
Chebrolu Hanumaiah Institute of Pharmaceutical Sciences, Chowdavaram, Guntur, Andhra Pradesh, 522019, India
Ramya Krishna Nakkala
Institute of Pharmacy and Technology, Salipur, Cuttack, Odisha, India
Bhabani Shankar Nayak
Department of Medicinal Products Medical Writing, Scientific Writing Services, Freyr Global Regulatory Solutions & Services, Hitech City, Gachibowli, Phoenix SEZ, Hyderabad, Telangana, 500081, India
Balaji Maddiboyina
You can also search for this author in PubMed Google Scholar
Contributions
BM involved in conceptualization, data curation, supervision, writing–original draft and review & editing; HR took part in supervision, writing—review & editing; R involved in data curation, conceptualization, supervision; SC involved in project administration, validation; SK took part in investigation, supervision; RN involved in data curation, supervision, writing—original draft; BN took part in supervision, writing—review & editing.
Corresponding author
Correspondence to Balaji Maddiboyina .
Ethics declarations
Ethics approval and consent to participate.
Not applicable.
Consent for publication
Competing interests.
The authors declare that they have no known competing financial interests or personal relationships that could have appeared to influence the work reported in this paper.
Additional information
Publisher's note.
Springer Nature remains neutral with regard to jurisdictional claims in published maps and institutional affiliations.
Rights and permissions
Open Access This article is licensed under a Creative Commons Attribution 4.0 International License, which permits use, sharing, adaptation, distribution and reproduction in any medium or format, as long as you give appropriate credit to the original author(s) and the source, provide a link to the Creative Commons licence, and indicate if changes were made. The images or other third party material in this article are included in the article's Creative Commons licence, unless indicated otherwise in a credit line to the material. If material is not included in the article's Creative Commons licence and your intended use is not permitted by statutory regulation or exceeds the permitted use, you will need to obtain permission directly from the copyright holder. To view a copy of this licence, visit http://creativecommons.org/licenses/by/4.0/ .
Reprints and permissions
About this article
Cite this article.
Maddiboyina, B., Roy, H., Ramaiah, M. et al. Methicillin-resistant Staphylococcus aureus : novel treatment approach breakthroughs. Bull Natl Res Cent 47 , 95 (2023). https://doi.org/10.1186/s42269-023-01072-3
Download citation
Received : 16 March 2023
Accepted : 20 June 2023
Published : 30 June 2023
DOI : https://doi.org/10.1186/s42269-023-01072-3
Share this article
Anyone you share the following link with will be able to read this content:
Sorry, a shareable link is not currently available for this article.
Provided by the Springer Nature SharedIt content-sharing initiative
- Antimicrobial
- Antibacterial
- Drug delivery
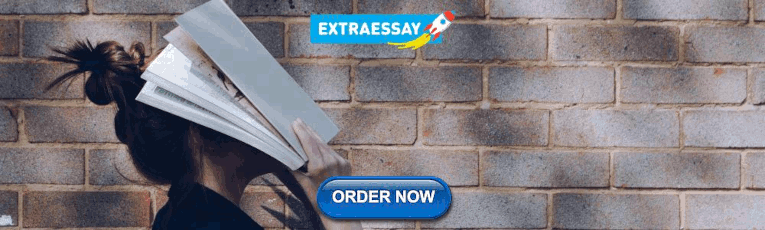
IMAGES
VIDEO
COMMENTS
Abstract. Methicillin-resistant Staphylococcus aureus (MRSA) is one of the most successful modern pathogens. The same organism that lives as a commensal and is transmitted in both health-care and community settings is also a leading cause of bacteraemia, endocarditis, skin and soft tissue infections, bone and joint infections and hospital ...
Methicillin-resistant Staphylococcus aureus (MRSA) is a major pathogen both within hospitals and in the community. In this Review, Fowler and colleagues provide an overview of basic and clinical ...
2. Emergence and types of MRSA. First time this bacteria was isolated from pus sample and given the name of "S. aureus" in 1881 (Ogston, 1881).Before the availability of penicillin, which was discovered by Radetsky (1996), an increased number of deaths were reported due to S. aureus infection that reached 90% case fatality rate which persisted up-to 19th century (Jevons, 1961).
PDF | On Jan 1, 2015, Hafsat Ali Grema and others published Methicillin resistant Staphyloccus aureus (MRSA): a review | Find, read and cite all the research you need on ResearchGate
In this Review , we provide an overview of basic and clinical MRSA research and summarize the expansive body of literature on the epidemiology , transmission, genetic diversity , evolution ...
Abstract. Methicillin-resistant Staphylococcus aureus (MRSA) is one of the most successful modern pathogens. The same organism that lives as a commensal and is transmitted in both health-care and community settings is also a leading cause of bacteraemia, endocarditis, skin and soft tissue infections, bone and joint infections and hospital ...
Download PDF. Download PDF. ... management and clinical effect of MRSA, focusing on HA-MRSA, and discusses future research priorities. ... This paper reveals how different S. aureus lineages have ...
The national estimated number of MRSA infections in the US, stratified by infection setting. Adapted from data reported by the Center for Disease Control and Prevention [27] and Dantes et al. [28].
An overview of basic and clinical MRSA research is provided and the expansive body of literature on the epidemiology, transmission, genetic diversity, evolution, surveillance and treatment of MRSA is explored. Methicillin-resistant Staphylococcus aureus (MRSA) is one of the most successful modern pathogens. The same organism that lives as a commensal and is transmitted in both health-care and ...
Following the spread of MRSA, glycopeptides (usually vancomycin and more recently teicoplanin) have become the mainstay of treatment for MRSA infections. 14 As vancomycin is commonly used for the treatment of MRSA infections, it has resulted in the development of vancomycin-intermediate S. aureus and vancomycin-resistant S. aureus. Vancomycin-
MRSA infections; however, there is a steady increase in com-munity-acquired MRSA infections, which poses challenges, particularly in densely populated countries like India. Further, the economic burden associated with the cost of treatment, long-term hospitalization, and the psychological stress considerably impact the healthcare systems across all
The Genomic level research of MRSA MGEs is a revealer complication of MRSA progression, where the spread, acquisition, and waste of the specific MGEs differ over time, likely affected via ...
Methicillin-resistant Staphylococcus aureus (MRSA) is associated with difficult-to-treat infections and high levels of morbidity. Manual practitioners work in environments where MRSA is a common acquired infection. The purpose of this review is to provide a practical overview of MRSA as it applies to the manual therapy professions (eg, physical ...
MRSA colonization is an important risk factor for subsequent infection in this population. Quasi-experimental studies have shown that decolonization can reduce MRSA infections during endemic and outbreak settings. Targeted and universal decolonization approaches have both been successfully used to reduce MRSA in this population.
A 2020 article revealed that MRSA commonly affects the skin and soft tissues. Bacteremia owing to S. aureus has been associated with 15% to 60% mortality rates. MRSA can also affect children to adults with no age restriction. MRSA bacteremia may lead to sudden death when there is no rapid diagnosis and treatment.
Methicillin-Resistant Staphylococcus aureus (MRSA) Gram-positive cocci bacteria that form clusters under gram staining. Approximately 30% of people are colonized with S. aureus in their nose. MRSA is coagulase-positive. Some MRSA are resistant to almost all antibiotics related to penicillin/beta-lactams. Oxacillin is commonly used to detect ...
Methicillin-resistant Staphylococcus aureus (MRSA) is an infection seen in many hospitals. It is a type of bacteria that are especially prevalent in hospitals and can cause severe sickness and death (Turner et al. 2019).According to recent prevalence studies, the incidence of MRSA is on the rise in developed countries, both in healthcare settings and in the community (Wan et al. 2021; Maddina ...
Since its initial description in the 1960s, methicillin-resistant Staphylococcus aureus (MRSA) has developed multiple mechanisms for antimicrobial resistance and evading the immune system, including biofilm production. MRSA is now a widespread pathogen, causing a spectrum of infections ranging from superficial skin issues to severe conditions like osteoarticular infections and endocarditis ...
PDF | Methicillin-resistant Staphylococcus aureus (MRSA) is a major human pathogen and a historically emergent zoonotic pathogen with public health and... | Find, read and cite all the research ...
Activity C: ELC Prevention Collaboratives. John Jernigan, MD, MS. Alex Kallen, MD, MPH. Division of Healthcare Quality Promotion. Centers for Disease Control and Prevention. Draft - 1/19/10 ---- Disclaimer: The findings and conclusions in this presentation are those of the authors and do not necessarily represent the official position of the ...
Introduction. Methicillin-resistant Staphylococcus aureus (MRSA) is considered as a historic emergent zoonotic pathogen with public health and veterinary importance.S. aureus causes serious problems in both humans and animals and could resist adverse environmental conditions such as sunlight and desiccation. 1, 2 The bacterium could invade the skin, mucous membranes and internal organs causing ...
In the 1980s and 1990s, the epidemic of MRSA spread across developed countries due to the rise of new clones. A study taken by Hetem and coworkers recommended that HA-MRSA clones tend to transfer at higher rates between hospitalized patients [].In 2008, over 380,000 HA-MRSA infections were acquired in European Union (EU) member states, Iceland, and Norway.
View PDF; Review Papers. ... select article Research progress of fullerenes and their derivatives in the field of PDT. ... select article Identification of CH<sub>2</sub>-linked quinolone-aminopyrimidine hybrids as potent anti-MRSA agents: Low resistance potential and lack of cross-resistance with fluoroquinolone antibiotics.
PDF | On Oct 20, 2018, Asinamai Athliamai Bitrus and others published Staphylococcus aureus: A Review of Antimicrobial Resistance Mechanism | Find, read and cite all the research you need on ...