- Our Program Divisions
- Our Three Academies
- Government Affairs
- Statement on Diversity and Inclusion
- Our Study Process
- Conflict of Interest Policies and Procedures
- Project Comments and Information
- Read Our Expert Reports and Published Proceedings
- Explore PNAS, the Flagship Scientific Journal of NAS
- Access Transportation Research Board Publications
- Coronavirus Disease 2019 (COVID-19)
- Diversity, Equity, and Inclusion
- Economic Recovery
- Fellowships and Grants
- Publications by Division
- Division of Behavioral and Social Sciences and Education
- Division on Earth and Life Studies
- Division on Engineering and Physical Sciences
- Gulf Research Program
- Health and Medicine Division
- Policy and Global Affairs Division
- Transportation Research Board
- National Academy of Sciences
- National Academy of Engineering
- National Academy of Medicine
- Publications by Topic
- Agriculture
- Behavioral and Social Sciences
- Biography and Autobiography
- Biology and Life Sciences
- Computers and Information Technology
- Conflict and Security Issues
- Earth Sciences
- Energy and Energy Conservation
- Engineering and Technology
- Environment and Environmental Studies
- Food and Nutrition
- Health and Medicine
- Industry and Labor
- Math, Chemistry, and Physics
- Policy for Science and Technology
- Space and Aeronautics
- Surveys and Statistics
- Transportation and Infrastructure
- Searchable Collections
- New Releases
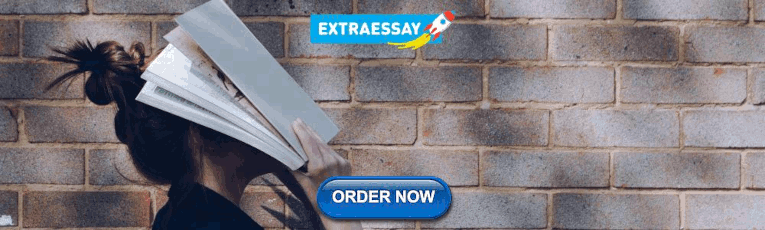
Biology and Life Sciences » Animals, Plants and Other Organisms
- Search Within This Topic
- Browse by Subtopic
- View All Topics
- Animals, Plants and Other Organisms
- Biodiversity
- Biotechnology
- Laboratory Animal Research
- Policy, Reviews and Evaluations
Viewing 1 - 10 of 168 books in Animals, Plants and Other Organisms
Animal Welfare Challenges in Research and Education on Wildlife, Non-Model Animal Species and Biodiversity: Proceedings of a Workshop (2022)
A Research Strategy to Examine the Taxonomy of the Red Wolf (2020)
Evaluation of Applications to Carry Out Research to Determine the Taxonomy of Wild Canids in the Southeastern United States (2020)
Evaluating the Taxonomic Status of the Mexican Gray Wolf and the Red Wolf: Spanish-Language Summary (2019)
Evaluating the Taxonomic Status of the Mexican Gray Wolf and the Red Wolf (2019)
Revisiting Brucellosis in the Greater Yellowstone Area (2020)
Approaches to Understanding the Cumulative Effects of Stressors on Marine Mammals (2017)
New Insights into Microbiome Study for Environmental Health: Proceedings of a Workshop—in Brief (2016)
The Role of Clinical Studies for Pets with Naturally Occurring Tumors in Translational Cancer Research: Workshop Summary (2015)
Field Guide to Brazil (1960)
Types of publications.
Proceedings: Proceedings published by the National Academies of Sciences, Engineering, and Medicine chronicle the presentations and discussions at a workshop, symposium, or other event convened by the National Academies. The statements and opinions contained in proceedings are those of the participants and are not endorsed by other participants, the planning committee, or the National Academies.
Consensus Study Reports: Consensus Study Reports published by the National Academies of Sciences, Engineering, and Medicine document the evidence-based consensus on the study’s statement of task by an authoring committee of experts. Reports typically include findings, conclusions, and recommendations based on information gathered by the committee and the committee’s deliberations. Each report has been subjected to a rigorous and independent peer-review process and it represents the position of the National Academies on the statement of task.
Rapid Expert Consultation: Rapid Expert Consultations published by the National Academies of Sciences, Engineering, and Medicine are authored by subject-matter experts on narrowly focused topics that can be supported by a body of evidence. The discussions contained in rapid expert consultations are considered those of the authors and do not contain policy recommendations. Rapid expert consultations are reviewed by the institution before release.
- Search Menu
- Advance articles
- Author Guidelines
- Open Access
- About Integrative and Comparative Biology
- About the Society for Integrative and Comparative Biology
- Editorial Board
- Advertising and Corporate Services
- Journals Career Network
- Self-Archiving Policy
- Dispatch Dates
- Journals on Oxford Academic
- Books on Oxford Academic
Article Contents
Introduction, separation: why and how, genesis of the symposium, complementary insights from studies of animals and plants, conclusion: paraphrasing krogh and dobzhansky, plant and animal physiological ecology, comparative physiology/biochemistry, and evolutionary physiology: opportunities for synergy: an introduction to the symposium 1.
- Article contents
- Figures & tables
- Supplementary Data
Martin E. Feder, Plant and Animal Physiological Ecology, Comparative Physiology/Biochemistry, and Evolutionary Physiology: Opportunities for Synergy: An Introduction to the Symposium, Integrative and Comparative Biology , Volume 42, Issue 3, July 2002, Pages 409–414, https://doi.org/10.1093/icb/42.3.409
- Permissions Icon Permissions
Both plant biologists and animal biologists seek to understand how their focal organisms have evolved to interact with the environment. Despite this similarity in goals, the differing biology of plants and animals as well as other factors have led these scientific communities to diverge. Scientific discoveries that have occurred in each community in relative isolation may advance progress in the other community and set the stage for broad scientific syntheses. The accompanying papers, summarized herein, exemplify such discoveries, and collectively argue that the plant and animal ecophysiological communities have much to gain from improved cooperation and communication.
Adaptation to the environment is a major theme in modern biology, and both plant and animal biologists have formed scientific communities around this theme. Both communities have interests in the mechanisms that enable their focal organisms to persist in natural environments, how these mechanisms enable persistence ( i.e., affect fitness) in the wild, and how evolution originated and maintains these mechanisms. Both communities study multiple levels of biological organization, from the molecule to the ecosystem, and both examine genotype and phenotype. Both communities might identify themselves as physiological ecologists, ecological physiologists, evolutionary physiologists, or environmental physiologists. Despite this similarity in outlook, scope, and objective, however, these plant biologist and animal biologist communities are often separate. At the level of scientific societies in the USA, the relevant animal biologists might join the Section of Comparative Physiology and Biochemistry of the Society for Integrative and Comparative Biology or the American Physiological Society, whereas the corresponding plant biologists might join the Physiological Ecology Section of the Ecological Society of America or the American Society for Plant Physiology. The two communities might also publish in different journals, attend different conferences, and otherwise distance themselves intellectually from one another. If this separation signals only the differing orientation of the two communities, it is an unfortunate but necessary consequence of the prevailing pressures towards specialization in the modern life sciences. By contrast, if this separation deprives each community of ideas, approaches, techniques, and relevant findings generated by the other, it then impedes scientific progress. The major purpose of the papers assembled here is to argue that this separation deprives each community of important benefits that could derive from better communication if not integration.
Clearly, the commitment of individuals or entire fields exclusively to botanical and zoological models is not obligatory. Darwin himself worked on both plant and animal material, and the rediscovery of Mendel's work on plants rapidly pervaded both plant and animal communities. Both plant and animal biologists contributed to the founding and development of ecology, and both ( e.g., Ledyard Stebbins, Theodosius Dobzhansky, George Simpson, Ernst Mayr) helped consolidate the New Evolutionary Synthesis. A few exceptional individuals ( e.g., Per Scholander [ Scholander, 1978 ]) made seminal contributions to both plant and animal ecophysiology, and others ( e.g., Nevo [1995] ) have similar goals in terms of evolutionary biology. One mechanistic/evolutionary discipline, comparative biomechanics, seems to investigate plant and animal materials with equal enthusiasm, and another, plant-animal interactions ( Schultz, 2002 ), depends on it.
But the mainstreams of the fields did diverge, and often for sound reasons. First, the modal plant differs from the modal animal. (As is immediately obvious, few attributes are unique to plants or animals: movement and behavior, sessile habits, photosynthesis, and tree-like morphology, for example, characterize some plants and some animals. Both kingdoms exhibit effective mechanisms for long-range dispersal in space and time [see below].) One especially consequential difference is in capacity for behavior; the modal animal can better escape from harmful or lethal environments than the modal plant ( Huey, 2002 ). In terms of mechanism, this difference leads to research emphasizing motility, control of motility, and metabolic support of activity in animals; and on resistance to stress or phenotypic plasticity in plants. In terms of population biology and evolution, Bradshaw (1970) suggests some typical differences: (a) plants are subject to stronger selection pressures than animals; (b) selection is more constant in direction in plants than in animals; (c) plant species comprise populations of smaller geographic size than those of animal species, and thus intraspecific variation in environmental regime will be greater in plant species than in animal species; (d) consequently, plant populations vary more in the direction of selection than animal species; (e) less gene flow among plant populations than among animal populations; (f) greater resemblance between the habitats of parents and offspring in plants; (g) less replacement of parents by offspring because of perenniation in plants; and (h) in response to environmental change, the entire breeding system may change more rapidly in plants than in animals. Huey (2002) explores these predictions in detail. Thus, as the focal or model organisms typically differ in substantial ways, the communities that study them may lack common foci.
A second reason for separate communities of plant and animal biologists is that the differing characteristics of typical subjects give rise to differing experimental technologies, laboratory husbandry procedures, and other logistical issues. As Huey (2002) observes, while the two communities might each focus on phenotypic plasticity, plant investigators routinely do so in terms of soil, light, and CO 2 while animal investigators routinely work on temperature and oxygen. Comparing the outcomes of this work becomes difficult. Thus, at a scientific meeting, a plant biologist may find sharing “the tools of the trade” with an animal biologist to be counterproductive (and vice versa).
A third reason for the separation may be that the animal community has undergone strong societal pressures to discover the basis and cure of human disease, and the plant community has not. Often these pressures have taken the form of massive funding from the U.S. National Institutes of Health and non-governmental biomedical organizations, which has led both individual scientists and academic administrators to eschew more broadly biological research in favor of biomedical relevance. One negative consequence in the USA was a decline in botany departments and marginalization of plant sciences (which must have encouraged resentment of animal scientists). One positive consequence may have been that the plant biologist community, less needing to relate its science to human disease, was freer to focus on diverse study systems and biological problems than the animal biologist community. Only recently has the plant biologist community converged upon a model organism ( Arabidopsis ) similar to those upon which the animal biologist/biomedical communities have converged ( Drosophila , mice and rats, yeast, E. coli , and recently C. elegans and zebrafish, among others). Another difference is that the exploitation of model organisms in the animal biologist/biomedical communities created a schism within this community (for an autobiographical account, see Wilson [1994 ]). On one side were biomedically-oriented scientists, often using model systems, often working at the molecular and cellular levels with experimental genetics as a principal tool, and often eschewing biological diversity in hopes of discovering the principles underlying the common features of living things. On the other side were “comparative” or non-biomedical scientists, often working at the organ, organismal or population level, and often eschewing model systems to discover the principles underlying biological diversity. The plant biology community escaped much of the suffering due to this schism. Although the schism has healed or is currently healing in the animal biologist community in the post-genomic era, it may be opening in the plant biologist community with the ever-increasing emphasis of Arabidopsis and the proliferation of agricultural plant genomes.
Whatever the causes of separation, the communities, once separate, developed along independent pathways and diverged. Each community fostered distinctive (but largely distinct) scientific accomplishments. Some recent book titles in plant physiological ecology (see http://www.botany.duke.edu/jackson/ecophys/books/index.html ) exemplify major themes in that community, including global climate change, atmospheric levels of carbon dioxide and trace gases, scaling from the individual plant to entire communities and ecosystems if not to the entire biosphere, abiotic stress in general and that of particular environments, and variation in metabolic pathways (C3 and C4): Plants in Changing Environments: Linking Physiological , Population , and Community Ecology ( Bazzaz, 1996 ); Plant Resource Allocation ( Bazzaz and Grace, 1997 ); Arctic Ecosystems in a Changing Climate: An Ecophysiological Perspective ( Chapin, 1992 ); Scaling Physiological Processes: Leaf to Globe ( Ehleringer and Field, 1993 ); Stable Isotopes and Plant Carbon-Water Relations ( Ehleringer et al. , 1993 ); Carbon Dioxide and Terrestrial Ecosystems ( Koch and Mooney, 1996 ); Carbon Dioxide , Populations , and Communities ( Körner and Bazzaz, 1996 ); Water Relations of Plants and Soils ( Kramer and Boyer, 1995 ); Carbon Dioxide and Environmental Stress ( Luo and Mooney, 1999 ); Terrestrial Global Productivity ( Mooney and Saugier, 2000 ); Response of Plants to Multiple Stresses ( Mooney, 1991 ); Physiology of Plants Under Stress: Abiotic Factors ( Nilsen and Orcutt, 1996 ); Physicochemical and Environmental Plant Physiology ( Nobel, 1999 ); Physiology of Plants Under Stress: Soil and Biotic Factors ( Orcutt and Nilsen, 2000 ); Landscape Function and Disturbance in Arctic Tundra ( Reynolds and Tenhunen, 1996 ); C4 Plant Biology ( Sage and Monson, 1999 ); Trace Gas Emissions by Plants ( Sharkey et al. , 1991 ); and Invasive Species in a Changing World ( Mooney and Hobbs, 2000 ). Interestingly, “evolution,” “adaptation,” and “molecular” are entirely absent from these titles, but are common in the counterpart animal literature ( Feder et al. , 2000 ). Although both communities have interests in stress ( e.g., from authors working primarily with animals, see Extreme Environmental Change and Evolution [ Hoffmann and Parsons, 1997 ] and Environmental Stress , Adaptation , and Evolution [ Bijlsma and Loeschcke, 1997 ]), the remainder of the plant themes (including “ecology” itself) are typically rare in the counterpart animal literature. As the accompanying papers and many individual research programs demonstrate, these differences neither are obligatory nor reflect disinterest of each community in the other's themes. Rather, just as diverging populations may by chance accumulate very different characteristics once they have speciated, the two communities happen to have developed in different directions.
The themes not developed in each community represent obvious opportunities for growth, but have done so for some time. The confluence of several events now makes the potential for cross-fertilization more evident. One is that general molecular biological techniques and genomics are increasingly permeating every discipline and scientific community. At the laboratory bench, plant and animal biologists are increasingly doing the same thing; in the scientific literature as well, questions and paradigms are increasingly converging as a result. Another event is the realization that cross-taxon communities and programs may have real advantages, economies of scale, and greater scientific strength through diversity. This realization has led to corresponding reorganizations and redefinition of missions. For example, when the National Science Foundation reorganized its programs in the 1990s, first as “Functional and Physiological Ecology” and then as “Ecological and Evolutionary Physiology,” it combined both plant and animal biology in this program, departing from its previous practice of separating the two. Also in the 1990s, the American Society of Zoologists became the Society for Integrative and Comparative Biology. A final event making this symposium timely is that the increasing dominance of Arabidopsis as a model system in the plant physiology community is leading non- Arabidopsis plant physiologists to realize that they may have much in common intellectually with non-model organism animal physiologists.
This confluence (and acquaintances formed when plant and animal biologists began to serve on a common National Science Foundation panel) led representatives of both the plant and animal communities to ponder whether closer ties at the level of scientific societies would be beneficial. This process culminated in two events. First, officers and representatives of the largely-zoological Society for Integrative and Comparative Biology and the largely-botanical Physiological Ecology Section of the Ecological Society of America organizing a workshop, entitled “Towards Potential Synergies of Plant and Animal Ecophysiology,” at the Ecological Society's annual meeting in August 2000. Second, these same two groups co-sponsored the symposium whose record is published here, entitled “Plant and animal physiological ecology, comparative physiology/biochemistry, and evolutionary physiology: opportunities for synergy” at the annual meeting of the Society for Integrative and Comparative Biology in January 2001. A grant from the Ecological and Evolutionary Physiology Program of the National Science Foundation supported both events.
The intent of the present symposium was two-fold. First, it was an experiment in social engineering. Just as the formation of a joint plant-animal Program at NSF led to interactions among plant and animal panelists that would not otherwise have occurred, this symposium was intended as a substrate for unprecedented interactions among plant and animal biologist symposiasts, members of the audience, and attendees of the SICB meeting. The outcome of this experiment is presently unknown. A second intent was, through the symposium presentations and the corresponding manuscripts published here, to exemplify common interests, paradigms, approaches, findings, principles, and opportunities for future scientific synergy between plant and animal scientists investigating organism-environment interactions. Of the many possible topics on which plant and animal investigators can provide complementary insights, the following papers touch on four.
Global climate change
Past or ongoing global climate change, whether natural or anthropogenic, can elucidate the physiology-environment interactions critical for persistence, extinction, or expansion of existing populations and species, and evolutionary changes in their traits. Predicting (if not mitigating) the biological impact of future climate change motivates this same understanding. Accordingly, global climate change has become a hot topic among both plant and animal ecophysiologists.
The accompanying paper by Ehleringer et al. (2002) is a striking example of the “Law of Unintended Consequences” in the context of global climate change. It suggests that past decreases in atmospheric CO 2 led to multiple independent evolutions of plants with C3 photosynthesis into plants with C4 photosynthesis, which subsequently proliferated in warm climates and expanded their ranges into higher latitudes during interglacial periods. The morphological changes in leaf structure to support C4 photosynthesis and the metabolic products/by-products of C4 photosynthesis in turn dramatically affected the nutritional quality of plants. This decreased nutritional quality in turn may have affected the diversity of mammalian herbivores; indeed, the expansion of C4-dominated ecosystems 6–8 Ma b.p. coincided with massive faunal change. Because the predominance of C3 and C4 species appears to be a joint function of atmospheric CO 2 levels and temperature among other variables, future generations could witness major changes in C4 abundance, with corresponding effects on herbivores.
As Porter et al. (2002) demonstrate, plants can influence the distribution and abundance of animals not only because animals eat them, but also because they determine the microclimates that animals experience. This demonstration exploits biophysical models of energy flux coupled with molar balance models of mass flux and GIS-based information on climate, topography, and vegetation. The models predict that for large mammals such as elk, radiative heat exchange with a forested canopy (instead of a clear night sky) is tantamount to a significant savings in energy that would otherwise be expended in thermoregulation. In other words, the loss of conifer needles due to forest fires may increase the daily energy requirement of elk by 20–25%. In a second example, Porter et al. (2002) calculate that exclusion of sunlight by vegetation may significantly reduce the temporal overlap of a basking ectothermic predator (rattlesnake) with its endothermic prey (ground squirrels). Thus, these models can reveal unsuspected but significant interactions among climate, terrain, vegetation, and animals.
Sensing and signaling
At one time, biomedical significance, the dramatic nature of animal responses to environmental stimuli, and the conspicuousness of nervous and endocrine systems in animals led studies of extracellular and intracellular signaling to advance more rapidly in animals than in plants. Since then, however, plant biologists have been making equally rapid advances, revealing signaling mechanisms that are comparable to animals' in many ways. For example, the chemical structures of jasmonic acid and its derivatives, major signaling molecules of plants, and eicosanoids such as prostaglandins in animals, are remarkably similar, suggesting either evolutionary homology or convergence in their use ( Schultz, 2002 ). So similar are these and other signaling mechanisms, in fact, that either kingdom may be able to exploit the other, as Schultz (2002) suggests. In one case that Schultz (2002) reviews, caterpillar regurgitant contains a signaling compound so similar to the plant's own that the corresponding plant signaling pathway is activated, leading to release of volatiles that attract caterpillar parasitoids. Alternatively, many insects synthesize plant cytokinins, which they administer to cause plants to form structures that protect the insect or its offspring ( i.e., galls). Thus, Schultz (2002) proposes that evolution has produced biochemical counterparts of much of modern warfare in plant and animal combatants: stealth, signal jamming, outright biowarfare, espionage, germ warfare, and signaling third parties to attack one's enemies.
Their ongoing warfare notwithstanding, plants and animals have common enemies, which include physical limitations and environmental conditions that may disrupt the normal supply or excretion of the respiratory gases, CO 2 and O 2 . As several of the accompanying papers ( Ehleringer et al. , 2002 ; Lutz and Prentice, 2002 ; Sage, 2002 ) discuss, atmospheric concentrations of both gases have fluctuated dramatically during Earth's history, and many organisms (or parts thereof) that are typically normoxic and normocapnic temporarily experience excesses or shortages of these gases. Through mechanisms that are becoming increasingly well-understood ( Lutz and Prentice, 2002 ; Sage, 2002 ), inappropriate gas exchange damages plant and animal cells. Accordingly, both plants ( Sage, 2002 ) and animals ( Lutz and Prentice, 2002 ) have evolved mechanisms for coping with acute change. These include acute adjustments to alter the transport of gas between organism and environment, “switches” that maintain productive metabolism despite changing gas levels, and activation of protective mechanisms and organized shutdown of cellular processes when all else fails. Many mechanistic issues remain unresolved, such as the identity and mechanism of the molecules that sense inappropriate gas levels and signal responses, and which genes undergo altered expression in response. An evolutionary issue is whether the acute response mechanisms that evidently can cope with current short-term variation in CO 2 and O 2 will also be able to cope with long-term anthropogenic changes in mean atmospheric gas levels ( Sage, 2002 ), or whether wholesale floral/faunal replacement will occur if these mechanisms cannot ( Ehleringer et al. , 2002 ).
Coping with stress in general
Individuals or populations may respond to environmental stress in at least 5 ways: behaviorally selecting favorable environments ( Huey, 2002 ), regulating the internal environment ( i.e., homeostasis), adjusting the impact of the environment on function ( i.e., phenotypic plasticity or acclimation), having a phenotype that is resistant to the impact of environmental stress, and/or evolutionary change in some or all of the preceding 4 alternatives. Many plants, animals, and other organisms combine these tactics in a stress-resistant stage of the life cycle (seed, spore, egg/embryo, pupa), often capable of prolonged stasis or diapause, which can disperse in space and/or time until benign conditions occur. This capacity may result in substantially different population and evolutionary dynamics than in organisms incapable of withdrawing from ongoing stress. For example, seed banks or egg banks may contain not only the offspring of the just-completed generation but those of up to thousands of generations past, allowing for remarkable mixtures of genotypes in the germinating generation. At the symposium in Chicago, Lawrence Venable discussed plant biologists' current view of these implications and ecological-evolutionary correlates of long-lived seeds, as well as his own work ( Venable and Brown, 1988 , 1993 ; Pake and Venable, 1995 , 1996 ; Venable and Pake, 1999 ; Clauss and Venable, 2000 ; Moriuchi et al. , 2000 ). Seed counterparts can be common in the animal kingdom; for example, 62% of crustacean species living in inland water habitats have prolonged egg diapause ( Hairston and Kearns, 2002 ). The parallel evolution of propagule dormancy/diapause in plants and animals provides several opportunities for researchers. First, independent tests of ecological and evolutionary predictions are readily possible ( Hairston and Kearns [2002] ; unlike for other aspects of phenotypic plasticity, in which plant and animal investigators typically study entirely different variables in different ways [ Huey, 2002 ]). Second, unlike with fossils, investigators can compare living organisms conceived many generations before present with their descendants and so directly study the historical evolution of non-morphological traits ( Feder et al. , 2000 ; Hairston and Kearns, 2002 ). A problem with so doing is that geological, limnological, or biological processes may mix the strata in which diapausing organisms are deposited, and obscure ancestor-descendant relationships. At least for zooplankton egg banks in lakes, this mixing may be insufficient to disrupt sediments completely, although the situation in terrestrial seed banks may be far more problematic ( Hairston and Kearns, 2002 ).
With respect to homeostasis, plants face several challenges that animals do not ( Holbrook et al. , 2002 ). First, they have few or no moving parts. They lack a heart and ventilatory, gastrointestinal, and thermoregulatory musculature, and thus must exploit other regulatory effectors. Second, much of a plant is dead, including the walls of cells and the entire xylem. Third, in terms of fluid balance, they take in and lose prodigious amounts of water by animal standards (each day a typical plant will lose ∼800 times as much as an animal of similar size ( Raven et al. , 1999 ), which must be replaced through dead tissue and without moving parts). As Holbrook et al. (2002) describe, plants replace this lost water by literally sucking it from the soil through their xylem, generating as much as −15 atmospheres of pressure in the process. Moreover, plants can modulate the conductance of these xylem vessels via active transport of solutes into them, which swells or deswells internal hydrogels. If the coherent water column ruptures ( i.e., is interrupted by a gas embolism), however, the result would be disastrous for plant water balance if not repaired. Holbrook et al. (2002) propose a remarkable 3-step mechanism for repair of embolisms. In the first step, living cells adjacent to the embolism actively pump ions into it, creating an osmotic gradient for water entry. Second, the geometry of pores (“pits”) connecting embolized xylem vessels to adjacent filled vessels creates a positive pressure forcing the gas in the embolus into solution. Third, reconnection to adjacent filled vessels must occur, but its mechanism currently awaits explanation.
Outright stress resistance, whether plastic or constitutive and in plants or animals, can be problematic because the features that make many biological molecules susceptible to stress ( i.e., weak bonds and conformational flexibility) are otherwise essential for function ( Hochachka and Somero, 1984 ; Somero, 1995 ). Desiccation has a particularly pernicious effect on cells via the cell membrane; even if the cell survives water loss, rehydration destroys the integrity of the membrane ( Crowe and Oliver, 2002 ). Despite this fact, some eukaryotes ( e.g., seeds and pollen of many plants, the resurrection plant [ Myrothamnus ], brine shrimp, yeast, some nematodes) can survive complete loss of their cellular water, as Crowe and Oliver (2002) describe. Remarkably, this ability appears to stem from a small number of common mechanisms involving accumulation of two disaccharides, trehalose and sucrose. In the first, when membranes pass through the gel-liquid crystalline phase transition, the contents of the cell may leak through them; trehalose and sucrose depress the temperature of the gel-liquid crystalline phase transition so that it does not occur at typical environmental temperatures. In another, even in dehydrated cells phospholipases may act on membranes to produce free fatty acids, which in turn destabilize the membrane; arbutin, a substance that some resurrection plants produce, inhibits phospholipases. To come full circle to biomedical relevance, described above as a potential contributor to the schism between plant and animal biologists: These lessons in stress tolerance learned from plants and fungi are now enabling the freeze-drying of blood platelets, which otherwise must be maintained in blood banks at room temperature and even then must be discarded after 5 days.
Although plants and animals clearly are different, each can elucidate principles of organism-environment interaction, biological mechanism, and evolutionary process and product that apply to both. For either the plant or the animal ecophysiological communities, to forgo the insights and advances produced by the other would be to squander a significant opportunity for scientific advancement. The Krogh Principle ( Krogh, 1929 ), often cited by animal physiologists ( Krebs, 1975 ), states: “For many problems there is an animal on which it can be most conveniently studied.” Perhaps the Krogh Principle needs revision; as written, plants (and, for the matter, members of all kingdoms) are “animals” too. But the interdependence of plant and animal studies is even more fundamental. As the most elementary of biology teaches, most plants and animals rely on one another for energy and mass fixation, and/or reproduction. As the accompanying papers and recent/forthcoming symposia on symbiosis in this journal exemplify particularly well, direct interactions of plants and animals have had a substantial impact on one another's evolution. Surely the investigation of plant and animal biology needs to mirror this evolution. Thus, to adapt the famous phrase of Dobzhansky (1973) , little in animal biology makes sense except in the light of plant biology, and vice versa.
From the symposium Plant/Animal Physiology presented at the Annual Meeting of the Society for Integrative and Comparative Biology, 3–7 January 2001, at Chicago, Illinois.
E-mail: [email protected]
This symposium, its publication in Integrative and Comparative Biology , and its predecessor workshop at the annual meeting of the Ecological Society of America have been supported by a grant from the National Science Foundation (IBN0097876), which also, through its formation of a joint plant-animal program in ecological and evolutionary physiology, set the stage for this symposium and supported much of the research described. Preparation of this manuscript was also supported by NSF Grant IBN9986158. Equally important was support from the Program Innovation Fund of the Society for Integrative and Comparative Biology (SICB). As described, this entire project was a collaborative effort of SICB, particularly the Division of Comparative Physiology and Biochemistry (DCPB), and the Physiological Ecology Section of the Ecological Society of America (PES-ESA). James Coleman (PES-ESA), Vincent Gutschick (PES-ESA), Arnold Bloom (a member of the American Society for Plant Physiology), Steven Hand (SICB-DCPB), and Dennis Bramble (SICB) all contributed to the organization and execution of the symposium and the ESA workshop. May Berenbaum (University of Illinois) contributed concluding remarks at the symposium.
Bazzaz , F. A. 1996 . Plants in changing environments: Linking physiological , population , and community ecology . Cambridge University Press, Cambridge.
Bazzaz , F. A. , and J. Grace. 1997 . Plant resource allocation . Academic Press, San Diego.
Bijlsma , R. , and V. Loeschcke.(eds.) 1997 . Environmental stress , adaptation , and evolution . Birkhäuser Verlag, Basel.
Bradshaw , A. D. 1970 . Some of the evolutionary consequences of being a plant. Evol. Biol , 5 25 -47.
Chapin , F. S. 1992 . Arctic ecosystems in a changing climate: An ecophysiological perspective . Academic Press, San Diego.
Clauss , M. J. , and D. L. Venable. 2000 . Seed germination in desert annuals: An empirical test of adaptive bet hedging. Am. Nat , 155 168 -186.
Crowe , J. H. , and A. E. Oliver. 2002 . Is there a single biochemical adaptation to anhydrobiosis? Integr. Comp. Biol , 42 497 -503.
Dobzhansky , T. 1973 . Nothing in biology makes sense except in the light of evolution. Am. Biology Teacher , 35 125 -129.
Ehleringer , J. R. , T. E. Cerling, and D. D. Dearing. 2002 . Atmospheric CO 2 as a global change driver influencing plant-animal interactions. Integr. Comp. Biol , 42 424 -430.
Ehleringer , J. R. , and C. B. Field. 1993 . Scaling physiological processes: Leaf to globe . Academic Press, San Diego.
Ehleringer , J. R. , A. E. Hall, and G. D. Farquhar. 1993 . Stable isotopes and plant carbon/water relations . Academic Press, San Diego.
Feder , M. E. , A. F. Bennett, and R. B. Huey. 2000 . Evolutionary physiology. Ann. Rev. Ecol. Syst , 31 315 -341.
Hairston , N. G. , Jr. , and C. M. Kearns. 2002 . Temporal dispersal: Ecological and evolutionary aspects of zooplankton egg banks and the role of sediment mixing. Integr. Comp. Biol , 42 481 -491.
Hochachka , P. W. , and G. N. Somero. 1984 . Biochemical adaptation . Princeton University Press, Princeton.
Hoffmann , A. A. , and P. A. Parsons. 1997 . Extreme environmental change and evolution . Cambridge University Press, Cambridge.
Holbrook , N. M. , M. A. Zwieniecki, and P. J. Melcher. 2002 . The dynamics of “dead wood”: maintenance of water transport through plant stems. Integr. Comp. Biol , 42 492 -496.
Huey , R. B. 2002 . Behavior as a buffer of environmental change. Integr. Comp. Biol , 42 415 -423.
Koch , G. W. , and H. A. Mooney. 1996 . Carbon dioxide and terrestrial ecosystems . Academic Press, San Diego.
Körner , C. , and F. A. Bazzaz. 1996 . Carbon dioxide , populations , and communities . Academic Press, San Diego.
Kramer , P. J. , and J. S. Boyer. 1995 . Water relations of plants and soils . Academic Press, San Diego.
Krebs , H. A. 1975 . The August Krogh principle: “For many problems there is an animal on which it can be most conveniently studied.”. J. Exp. Zool , 194 221 -226.
Krogh , A. 1929 . Progress of physiology. Am. J. Physiol , 90 243 -251.
Luo , Y. , and H. A. Mooney. 1999 . Carbon dioxide and environmental stress . Academic Press, San Diego, California.
Lutz , P. M. , and H. M. Prentice. 2002 . Sensing and responding to hypoxia: Molecular and physiological mechanisms. Integr. Comp. Biol , 42 463 -468.
Mooney , H. A. 1991 . Response of plants to multiple stresses . Academic Press, San Diego.
Mooney , H. A. , and R. J. Hobbs. 2000 . Invasive species in a changing world . Island Press, Washington, D.C.
Mooney , H. A. , and B. Saugier. 2000 . Terrestrial global productivity . Academic Press, San Diego.
Moriuchi , K. S. , D. L. Venable, C. E. Pake, and T. Lange. 2000 . Direct measurement of the seed bank age structure of a Sonoran Desert annual plant. Ecology , 81 1133 -1138.
Nevo , E. 1995 . Asian, African and European biota meet at Evolution Canyon, Israel—local tests of global biodiversity and genetic diversity patterns. Proc. Royal Soc. London , 262B 149 -155.
Nilsen , E. T. , and D. M. Orcutt. 1996 . The physiology of plants under stress: Abiotic factors . Wiley, New York.
Nobel , P. S. 1999 . Physicochemical & environmental plant physiology . Academic Press, San Diego.
Orcutt , D. M. , and E. T. Nilsen. 2000 . Physiology of plants under stress: Soil and biotic factors . Wiley, New York.
Pake , C. E. , and D. L. Venable. 1995 . Is coexistence of Sonoran Desert annuals mediated by temporal variability in reproductive success. Ecology , 76 246 -261.
Pake , C. E. , and D. L. Venable. 1996 . Seed banks in desert annuals: Implications for persistence and coexistence in variable environments. Ecology , 77 1427 -1435.
Porter , W. P. , J. L. Sabo, C. R. Tracy, O. J. Reichman, and N. Ramankutty. 2002 . Physiology on a landscape scale: Plant-animal interactions. Integr. Comp. Biol , 42 431 -453.
Raven , P. H. , R. F. Evert, and S. E. Eichhorn. 1999 . Biology of plants . W. H. Freeman: Worth Publishers, New York.
Reynolds , J. F. , and J. D. Tenhunen. 1996 . Landscape function and disturbance in arctic tundra . Springer, Berlin.
Sage , R. F. 2002 . How terrestrial organisms sense, signal, and respond to carbon dioxide. Integr. Comp. Biol , 42 469 -480.
Sage , R. F. , and R. K. Monson. 1999 . C|4 plant biology . Academic Press, San Diego.
Scholander , P. F. 1978 . Rhapsody in science. Ann. Rev. Physiol , 40 1 -7.
Schultz , J. C. 2002 . Shared signals and the potential for phylogenetic espionage between plants and animals. Integr. Comp. Biol , 42 454 -462.
Sharkey , T. D. , E. A. Holland, and H. A. Mooney. 1991 . Trace gas emissions by plants . Academic Press, San Diego.
Somero , G. N. 1995 . Proteins and temperature. Ann. Rev. Physiol , 57 43 -68.
Venable , D. L. , and J. S. Brown. 1988 . The selective interactions of dispersal, dormancy, and seed size as adaptations for reducing risk in variable environments. Am. Nat , 131 360 -384.
Venable , D. L. , and J. S. Brown. 1993 . The population-dynamic functions of seed dispersal. Vegetatio , 108 31 -55.
Venable , D. L. , and C. E. Pake. 1999 . Population ecology of Sonoran Desert annual plants. In R. H. Robichaux (ed.), The ecology of Sonoran Desert plants and plant communities, pp. 115–142. University of Arizona Press, Tucson.
Wilson , E. O. 1994 . Naturalist . Island Press/Shearwater Books, Washington, D.C.
Email alerts
Citing articles via.
- Recommend to your Library
Affiliations
- Online ISSN 1557-7023
- Print ISSN 1540-7063
- Copyright © 2024 The Society for Integrative and Comparative Biology
- About Oxford Academic
- Publish journals with us
- University press partners
- What we publish
- New features
- Open access
- Institutional account management
- Rights and permissions
- Get help with access
- Accessibility
- Advertising
- Media enquiries
- Oxford University Press
- Oxford Languages
- University of Oxford
Oxford University Press is a department of the University of Oxford. It furthers the University's objective of excellence in research, scholarship, and education by publishing worldwide
- Copyright © 2024 Oxford University Press
- Cookie settings
- Cookie policy
- Privacy policy
- Legal notice
This Feature Is Available To Subscribers Only
Sign In or Create an Account
This PDF is available to Subscribers Only
For full access to this pdf, sign in to an existing account, or purchase an annual subscription.
SPECIALTY GRAND CHALLENGE article
Plant biology research: what is next.
- Department of Plant and Microbial Biology, Program in Genetics, North Carolina State University, Raleigh, NC, United States
Plant biology is a key area of science that bears major weight in the mankind's ongoing and future efforts to combat the consequences of global warming, climate change, pollution, and population growth. An in-depth understanding of plant physiology is paramount to our ability to optimize current agricultural practices, to develop new crop varieties, or to implement biotechnological innovations in agriculture. The next-generation cultivars would have to withstand environmental contamination and a wider range of growth temperatures, soil nutrients and moisture levels and effectively deal with growing pathogen pressures to continue to yield well in even suboptimal conditions.
What are the next big questions in plant physiology, and plant biology in general, and what avenues of research should we be investigating and training students in for the next decade? As a plant scientist surrounded by like-minded individuals, I hear a lot of ideas that over time turn into buzz words, such as plant resilience, genotype-to-phenotype, data science, systems biology, biosensing, synthetic biology, neural networks, robustness, interdisciplinary training, new tool development, modeling, etc. What does it all mean and what are the main challenges that we should all be working on solving? Herein, I present my personal perspective on what the immediate questions and the biggest longer-term issues in plant science are. I suggest some themes and directions for future research in plant biology, some relatively obvious and some potentially unique, having been shaped by my own professional interests, experiences and the background in plant molecular genetics and physiology.
Integration, Packaging, Visualization and Interpretation of Existing OMICS and Genetic Data
For the past three decades, a lot of emphasis has been made on a small set of plant model organisms, primarily on Arabidopsis. There is no other plant on earth we know as much about as we do about this mustard weed. One clear need in the area of plant sciences is to make sense of the vast amount of descriptive phenotypic data that have been generated for this species and a handful of others—the transcriptome, metabolome, proteome, phenome, interactome, etc.—and the amazing genetic resources that have been built: mutants, transgenic lines and natural accession germplasm collections, tools and protocols, genomic sequences and other resources ( Koorneef and Meinke, 2010 ). Now, how do we organize these data into a series of integrated, comprehensive, user-friendly, cross-communicating databases that are easily accessible, searchable, trackable, and visual, with data that are downloadable and compatible with comparative analyses? How do we display the available data at a variety of scales, from the subcellular to the organismal and population level—think Google Earth but for an ecosystem or an agricultural field that allows you to zoom in and out to see the overview and the closeup—perhaps, by integrating and expanding existing initiative likes Plant Cell Atlas and ePlant ( Waese et al., 2017 ; Rhee et al., 2019 )? With the genome sequences of these select organisms in hand, often of multiple accessions of each, what can we learn about the genotype-to-phenotype relations? How can we use that knowledge to extrapolate the rules or patterns we discover in model organisms to species for which we have no experimental data beyond possibly a draft-quality genomic sequence and a few fragmentary phenotypic datasets? In other words, can the data obtained in reference organisms be leveraged to infer useful information relevant to a wide range of species of agricultural, ecological or, perhaps, ethnobotanical importance? Let's look into some examples of that.
Translational Research: Moving Foundational Discoveries From Models to Crops
It comes as no surprise that for the past 10–20 years the emphasis has been gradually shifting from Arabidopsis to non-model organisms, including crops and rare plant species. The key reason for that is the pressing need to move fast on crop improvement and plant conservation in light of the worlds' fast-growing population, climate change, pollution, habitat and agricultural land loss, and ever-increasing pathogen pressures. This shift of research focus is also steered by changing governmental policies and funders' priorities. To make the transition to studying crops and other non-models as smooth as possible, robust computational pipelines are needed that produce high-quality genome assemblies from combinations of short- and long-read sequences. In this regard, tackling the much more complex genomes of polyploid species presents an even greater challenge. With the genome sequences and high-quality assembles on hand, orthologous genes that have previously been studied only in reference organisms need to be tested for function in candidate processes in the non-model species of interest to determine what aspects of their function are conserved and what features are divergent. The key bottleneck in this process is, of course, the recalcitrance of many non-models to genetic transformation and plant regeneration ( Anjanappa and Gruissem, 2021 ). Thus, a major effort would need to be invested into new method development to improve the plant in vitro culturing, genetic transformation and regeneration pipelines, with the ectopic activation of morphogenesis genes like BABY BOOM, WUSCHEL, LEAFY COTYLEDON1 and 2 , and several others holding major promise for boosting the regeneration efficiency of otherwise recalcitrant plant species and cultivars ( Gordon-Kamm et al., 2019 ). Further optimization of genome editing technologies, including classical gene disruption through indels as well as more targeted gene edits via base- and prime-editing or homologous-recombination-based methods, should enable highly tailored manipulation of genes of interest. The foundational knowledge gained in both model and non-model organisms can then be leveraged by applied plant biologists and environmentalists in crop improvement and plant conservation.
Interpreting the Code
One aspect of experimental research we have become good at over the past 10 years is genome and transcriptome sequencing. The current challenge is to learn to infer what the sequence tells us about what a gene does and how it is regulated based on the code alone. Can we look at gene's genomic sequence and infer not only the gene function, but also the different levels of gene regulation, all from just the sequence without any additional experimentation? To elaborate on that distinction between function and regulation, we can already infer the likely function of an orthologous gene in a crop (previously studied in another species) based on the degree of conservation of its genomic sequence, and deduce, for instance, an enzymatic reaction a protein may catalyze, or a DNA element a transcription factor may bind, or a specific ion the channel may transport, or an array of ligands or other molecules a protein may interact with. What we cannot yet reliably do is to predict based on the gene sequence alone when and where the gene is transcribed and what environmental or developmental stimuli alter its expression, how stable its transcript is, what splicing patterns the transcript has in specific cell types or conditions, or what factors dictate these patterns, or how well the transcript is translated, how the protein folds, where in the cell the protein is targeted, what its half-life is, and so on. Can we someday look at the gene sequence and predict whether the gene is essential or what organ or tissues will be affected in the loss- or gain-of-function mutant, and what phenotype the mutant will show, all without having to run an experiment? Once we learn to do that for a diploid model plant, can the knowledge be translated to polyploids that may have a greater level of gene redundancy and potentially more cases of neofunctionalization? How do we gain that extraordinary power?
One of the critical components of the inferring-the-function or genotype-to-phenotype challenge will involve machine learning and neural network models, with the size and quality of the training datasets presenting as the likely bottleneck that would determine the accuracy of neural networks' predictions ( Ching et al., 2018 ). While the role of computational biologists in this endeavor would be to develop new algorithms or adapt existing pipelines and test the models, the irreplaceable function of experimental plant biologists in this effort will be to generate the most complete and robust datasets for model training. This inevitably brings us to the next big theme, data quality.
Data Quality: Standardization, Reliability, Robustness and Tracking
As experimental scientists, most if not all of us have had the negative experience of not being able to reproduce an important result (sometimes even our own) or confirm the identity of a material someone has shared with us (e.g., a strain, a plasmid, or a seed stock from a colleague or another lab). Issues with biological variation (e.g., differences in germination between seed batches), small sample size (due to prohibitive cost, time or material constraints, or other limitations), human error (suboptimal labeling nomenclature, poor tracking, inadequate record keeping, substandard experimental design, miscalculation, personnel changes, or outright sloppiness) or malfunctioning instrumentation (in many cases, due to the lack of funding or time to upkeep or upgrade the equipment) can all contribute to the limited reproducibility of experimental data or sample mix-up. Rarely is the wrongdoing intentional, but the consequences of these errors can be enormous. What can we do to minimize mistakes, standardize internal lab protocols and record keeping, and ultimately improve the reproducibility of published data? I would support a universal funder's mandate for detailed electronic note keeping (much like private companies require), automatic data backups and regular equipment upgrades, meticulous planning before an experiment is run (including developing a comprehensive sample labeling nomenclature, beyond the common 1, 2, 3), inclusion of universal controls (e.g., Arabidopsis Columbia accession included in every Arabidopsis experiment irrespective of what other germplasm is being tested), extensive sample replication, validation of the results at multiple steps in the process (like Sanger sequencing of construct intermediates), and other common-sense but often time-consuming practices (such as regrowing all genotypes side by side and using fresh seed stocks in an experiment to minimize seed batch effects, or resequencing every construct before donating it to the stock center or sharing it with others).
A different yet related constraint we often encounter in plant sciences is the inability to track and/or obtain the materials or datasets reported by other research groups or oftentimes even by prior members of one's own lab. To ensure the long-term availability and unrestricted access to published constructs, germplasm, omics datasets and other resources generated by the public sector, funding agencies should make it mandatory for all materials and data to be deposited in relevant stock centers, sequence repositories, etc. immediately upon publication. I often wonder whether this practice could be encouraged if one's scientific productivity and impact were to be evaluated not only by the number of papers published, but also by the number of stocks or datasets deposited and their usage by the community (e.g., the frequency of stock orders or data downloads). Publishers, on the other hand, should fully enforce the old rules that all submitted manuscripts must adhere to the established guidelines for proper scientific nomenclature (e.g., gene accession numbers, mutant names, or chemical structures) and include community access codes (e.g., gene identifiers, mutant stock numbers, Genbank accession codes, etc.) and detailed annotations for all materials and data utilized or generated in a study, with the compliance being a prerequisite for publication. These simple steps would reduce ambiguities, facilitate resource tracking, and make published materials and datasets universally available.
The extra effort invested into careful experiment planning, execution, record keeping, and making published materials and datasets trackable and accessible will undoubtedly lead to fewer but higher-quality research papers being published and ultimately save time and resources down the road. Of course, an external mandate for greater rigor and accountability would also mean the need for funding agencies to financially support the extra effort and develop ways to monitor the labs' adherence to the new stricter rigor and dissemination practices, but it is commonsense that in the long run it is cheaper to do the experiment right the first time around than waste years trying to reproduce or follow up on erroneous data or remaking the resource that has been generated previously.
Synthetic Biology
An exciting and highly promising area of sciences that plant biologists are starting to embrace more widely is synthetic biology. First, what is synthetic biology? To a plant biologist, it is a useful extension of classical molecular genetics that integrates basic engineering principles and aims to rebuild biology from the ground up. Traditionally, classically trained biologists approach learning about nature from top to bottom, much like a curious child trying to break a toy apart to see what it is made of. Synthetic biologists, vice versa, try to rebuild a functional system from its pieces to understand what its minimal required components are. In plant biology, we are still very far from being able to rebuild entire plants or plant cells from scratch, but we can reconstitute the pathways, e.g., those that we have previously studied in their native context, in a heterologous host cell, aka the chassis, or introduce simple gene regulatory circuits we have artificially built. Why would we want to do that? For one, to see if we can recreate the native behavior to ensure that we fully understand the pathway or the mechanism of regulation. In addition, this can be a useful endeavor from a practical perspective, as is the case in metabolic engineering, where a native or semi-synthetic biosynthetic pathway is expressed in a heterologous host (an intact plant or a cell suspension) to produce a valuable metabolite ( Lu et al., 2016 ; Birchfield and McIntosh, 2020 ), or in biosensing, where a synthetic genetic construct is introduced to turn the host into a bio-detector for a particular stimulus or ligand of interest, e.g., a metabolite ( Garagounis et al., 2021 ).
We do not fully comprehend what we cannot ourselves recreate. We may know, for example, that a gene is induced, for example, by heat stress, but that observation does not tell us anything about the developmental regulation of that gene, or what other biotic or abiotic factors control this gene's expression. An illustrative example of how limited our current knowledge is and how synthetic biology can help us to bypass the lack of comprehensive understanding is to try the following mental exercise. How would one go about conferring a desired pattern of expression to a gene of interest, so that the gene is transcribed, for example, only in a flower, in the anthers at a particular stage of flower development, and only in response to heat stress? If we are talking about a model organism, we can scavenge available transcriptomic data in hopes of finding a native gene with such a pattern, but chances are that most anther-enriched genes will be expressed elsewhere and/or will be regulated by stimuli other than the heat stress. With the vast amount of transcriptomic data and limited ChIP-seq, DAP-seq and chromatin availability data (ATAC-seq, DNase-seq, etc.), we still have no reliable ways to infer transcription patterns of a native gene across all tissues and conditions. A combination of bioinformatic analysis (to identify putative transcription factor binding sites based on sequence conservation) ( Zemlyanskaya et al., 2021 ), classical transgene promoter bashing (that involves building a series of transgenes with chunks of the promoter deleted or replaced in an effort to characterize the effect of these targeted DNA modifications on the expression of a reporter gene in a systematic manner) ( Andersson and Sandelin, 2020 ), and/or more recently, in planta promoter bashing via genome editing (i.e., generating targeted promoter modifications directly in the native genomic context) ( Pandiarajan and Grover, 2018 ) are often relied upon to identify regulatory cis -elements in the promoters of interest. However, these approaches will not be enough to identify the full array of the DNA cis -elements that dictate the spatiotemporal regulation of a gene of interest, but these strategies may be helpful at pinpointing some candidate cis -elements and experimentally validating which elements are required.
If a particular DNA element is experimentally shown to be necessary, let's say, for heat stress upregulation, the next step is to test if the element is sufficient. This could be done by building a tandem of these elements, making a synthetic proximal promoter and placing it upstream of a well-characterized core promoter like that of 35S to drive a reporter ( Ali and Kim, 2019 ). In the best-case scenario, if we are successful with finding an element that can confer heat-inducible expression to the reporter, we have no easy way of restricting this heat-activated expression to just the anthers, let alone at a specific stage of anther development. Even if we had another DNA element at hand that confers tissue-specific expression (in this example, in anthers), we have no straightforward way of implementing what computer scientists would view as the Boolean AND logic—to combine these DNA elements (e.g., in a single proximal promoter) in a manner that the transcription of the gene will now only be triggered specifically in anthers in response to heat, but not in any other conditions or tissues. Synthetic biology makes the implementation of that AND logic (and other types of Boolean logic gates) possible, e.g., through the use of heterodimeric transcription factors, with one monomer active in anthers (through the use of an anther-specific promoter) and another monomer expressed only in response to heat stress (through the use of a heat-regulated promoter) ( Figure 1 ). In this scenario, the full heterodimeric transcription factor would only be reconstituted in the anthers of heat-treated plants and will activate its target genes only in those flower tissues specifically under heat stress.
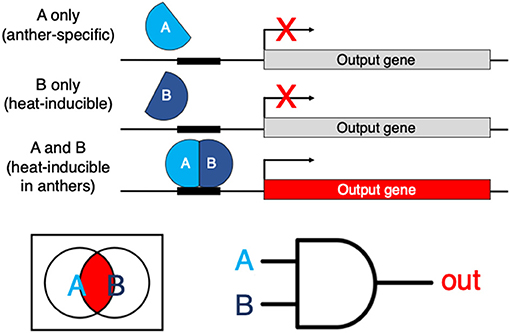
Figure 1 . An example of a hypothetical genetic Boolean logic AND gate. AB is a heterodimeric transcription factor. If subunit A is expressed in anthers and subunit B is inducible by heat, the full transcription factor is reconstituted only in heat-stressed anthers. The AND logic restricts the expression of the output gene of interest specifically to the tissues and conditions where/when both A and B are-co-expressed.
Thus, synthetic biology enables us to build genetic devices capable of controlling specific processes of interest despite the lack of the full mechanistic understanding of all the moving parts in those processes. In the near future, more and more plant biologists will adopt synthetic biology as a powerful way to bypass some of the technical bottlenecks in plant sciences. Who knows, someday futuristic concepts of a minimal plant genome and a minimal plant cell ( Yang et al., 2020 ) may even become a reality. How soon will we have a thorough enough understanding of plant molecular genetics and physiology, so that we can determine the minimal set of genes to make a functional plant that can stay alive in a single stable (optimal) environment? What would we need to add to the minimal system to make the plant now capable of responding to stress and thriving in less-than-optimal conditions? Although one would agree that we have a very long way before we can get there, it is not too early to start thinking about those more ambitious projects, while working on still very difficult but more achievable shorter-term goals where synthetic biology will play a central role, such as developing nitrogen-fixing cereal crops ( Bloch et al., 2020 ) or C4 rice ( Ermakova et al., 2020 ).
Other Directions and Concluding Remarks
Several other areas relevant to plant sciences will have paramount importance to our ability to propel plant biology research forward. Advanced automated high-throughput imaging and phenotyping will provide a more systematic, robust way to collect reliable morphometric data on a diversity of plant species in the lab, the greenhouse, and the field. New computational tool development and the implementation of novel experimental methods, along with the optimization and streamlining of existing tools and protocols, will remain the main driver of research progress, with single-cell omics approaches likely taking center stage for the next few years. Data science will play an even more predominant role given the vast amount of new data being generated and the need to handle and make sense of all that information. Systems-level approaches, mathematical modeling and machine learning will become a more integral part of plant biology research, enabling scientists to systematize and prioritize complex data and provide plant researchers with experimentally testable predictions.
If we want to see the breakthroughs we are making at the bench or in the field implemented in real-life products, we also need to work on shifting the public perception of biotechnologies. Critical steps toward rebuilding public trust in science include a greater understanding of the societal impacts of proposed innovations through collaboration with social scientists, the engagement of researchers with the science policy making process, and the active participation of all scientists (students, postdocs, technicians, faculty, industry professionals, etc.) in community outreach programs to make our work—and its implications—accessible to the general public. Lastly, one essential factor that would make the scientific advancements sustainable in the long run is a generous investment into the robust, trans-disciplinary training of the next generation of plant scientists. Our ability to create a welcoming environment for trainees from all backgrounds and paths of life would allow these students and postdocs to feel that their research team is their second family. Today's trainees are the ones who will be solving the world's pressing issues for years to come. Our ability to provide young scientists with the solid knowledge base and diverse skills would ensure that they are well equipped to take on the next big challenge.
Looking ahead, fundamental research on model organisms, applied work on crops, and conservation studies on rare plants will all continue to be of vital importance to modern plant biology. High-throughput inquiries and gene-specific projects done by mega-groups and small labs in state-of-the-art facilities or traditional field labs will all remain indispensable to the progress of plant sciences. In the end, addressing pressing societal issues like feeding the world's growing population and mitigating climate change ultimately rests on our ability as scientists to come together and harness the power of plants. Plant biology research is positioned to play a central role in this critical endeavor. It is an exciting and urgent time to be—or become—a plant scientist.
Author Contributions
The author confirms being the sole contributor of this work and has approved it for publication.
The work in the Stepanova lab is supported by the National Science Foundation grants NSF 1750006, NSF 1444561, NSF 1940829.
Conflict of Interest
The author declares that the research was conducted in the absence of any commercial or financial relationships that could be construed as a potential conflict of interest.
Publisher's Note
All claims expressed in this article are solely those of the authors and do not necessarily represent those of their affiliated organizations, or those of the publisher, the editors and the reviewers. Any product that may be evaluated in this article, or claim that may be made by its manufacturer, is not guaranteed or endorsed by the publisher.
Ali, S., and Kim, W. C. (2019). A fruitful decade using synthetic promoters in the improvement of transgenic plants. Front. Plant. Sci. 10:1433. doi: 10.3389/fpls.2019.01433
PubMed Abstract | CrossRef Full Text | Google Scholar
Andersson, R., and Sandelin, A. (2020). Determinants of enhancer and promoter activities of regulatory elements. Nat. Rev. Genet. 21, 71–87. doi: 10.1038/s41576-019-0173-8
Anjanappa, R. B., and Gruissem, W. (2021). Current progress and challenges in crop genetic transformation. J. Plant Physiol. 261:153411. doi: 10.1016/j.jplph.2021.153411
Birchfield, A. S., and McIntosh, C. A. (2020). Metabolic engineering and synthetic biology of plant natural products—a minireview. Curr. Plant Biol. 24:100163. doi: 10.1016/j.cpb.2020.100163
CrossRef Full Text | Google Scholar
Bloch, S. E., Ryu, M. H., Ozaydin, B., and Broglie, R. (2020). Harnessing atmospheric nitrogen for cereal crop production. Curr. Opin. Biotechnol. 62, 181–188 doi: 10.1016/j.copbio.2019.09.024
Ching, T., Himmelstein, D. S., Beaulieu-Jones, B. K., Kalinin, A. A., Do, B. T., Way, G. P., et al. (2018). Opportunities and obstacles for deep learning in biology and medicine. J. R. Soc. Interface 15:20170387. doi: 10.1098/rsif.2017.0387
Ermakova, M., Danila, F. R., Furbank, R. T., and von Caemmerer, S. (2020). On the road to C4 rice: advances and perspectives. Plant J. 101, 940–950. doi: 10.1111/tpj.14562
Garagounis, C., Delkis, N., and Papadopoulou, K. K. (2021). Unraveling the roles of plant specialized metabolites: using synthetic biology to design molecular biosensors. New Phytol. 231, 1338–1352. doi: 10.1111/nph.17470
Gordon-Kamm, B., Sardesai, N., Arling, M., Lowe, K., Hoerster, G., Betts, S., et al. (2019). Using morphogenic genes to improve recovery and regeneration of transgenic plants. Plants (Basel) 8. doi: 10.3390/plants8020038
Koorneef, M., and Meinke, D. (2010). The development of Arabidopsis as a model plant. Plant J. 61, 909–921. doi: 10.1111/j.1365-313X.2009.04086.x
Lu, X., Tang, K., and Li, P. (2016). Plant metabolic engineering strategies for the production of pharmaceutical terpenoids. Front. Plant. Sci. 7:1647. doi: 10.3389/fpls.2016.01647
Pandiarajan, R., and Grover, A. (2018). In vivo promoter engineering in plants: are we ready? Plant Sci. 277, 132–138. doi: 10.1016/j.plantsci.2018.10.011
Rhee, S., Birnbaum, K. D., and Ehrhardt, D. W. (2019). Towards building a plant cell atlas. Trends Plant Sci . 24, 303–310. doi: 10.1016/j.tplants.2019.01.006
Waese, J., Fan, J., Pasha, A., Yu, H., Fucile, J., Shi, R., et al. (2017). ePlant: visualizing and exploring multiple levels of data for hypothesis generation in plant biology. Plant Cell 29, 1806–1821. doi: 10.1105/tpc.17.00073
Yang, X., Medford, J. I., Markel, M., Shih, P. M., De Paoli, H. C., Trinh, C. T., et al. (2020). Plant biosystems design research roadmap 1.0. BioDesign Res. 2020:8051764. doi: 10.34133/2020/8051764
Zemlyanskaya, E. V., Dolgikh, V. A., Levitsky, V. G., and Mironova, V. (2021). Transcriptional regulation in plants: using omics data to crack the cis-regulatory code. Curr. Opin. Plant Biol. 63:102058. doi: 10.1016/j.pbi.2021.102058
Keywords: plant biology, plant physiology, synthetic biology, translational research, data reproducibility
Citation: Stepanova AN (2021) Plant Biology Research: What Is Next? Front. Plant Sci. 12:749104. doi: 10.3389/fpls.2021.749104
Received: 05 August 2021; Accepted: 06 September 2021; Published: 30 September 2021.
Edited and reviewed by: Joshua L. Heazlewood , The University of Melbourne, Australia
Copyright © 2021 Stepanova. This is an open-access article distributed under the terms of the Creative Commons Attribution License (CC BY) . The use, distribution or reproduction in other forums is permitted, provided the original author(s) and the copyright owner(s) are credited and that the original publication in this journal is cited, in accordance with accepted academic practice. No use, distribution or reproduction is permitted which does not comply with these terms.
*Correspondence: Anna N. Stepanova, atstepan@ncsu.edu
Disclaimer: All claims expressed in this article are solely those of the authors and do not necessarily represent those of their affiliated organizations, or those of the publisher, the editors and the reviewers. Any product that may be evaluated in this article or claim that may be made by its manufacturer is not guaranteed or endorsed by the publisher.
Thank you for visiting nature.com. You are using a browser version with limited support for CSS. To obtain the best experience, we recommend you use a more up to date browser (or turn off compatibility mode in Internet Explorer). In the meantime, to ensure continued support, we are displaying the site without styles and JavaScript.
- View all journals
- Explore content
- About the journal
- Publish with us
- Sign up for alerts
- Review Article
- Published: 27 October 2021
Plant and animal small RNA communications between cells and organisms
- Xuemei Chen ORCID: orcid.org/0000-0002-5209-1157 1 &
- Oded Rechavi 2 , 3
Nature Reviews Molecular Cell Biology volume 23 , pages 185–203 ( 2022 ) Cite this article
15k Accesses
68 Citations
152 Altmetric
Metrics details
Since the discovery of eukaryotic small RNAs as the main effectors of RNA interference in the late 1990s, diverse types of endogenous small RNAs have been characterized, most notably microRNAs, small interfering RNAs (siRNAs) and PIWI-interacting RNAs (piRNAs). These small RNAs associate with Argonaute proteins and, through sequence-specific gene regulation, affect almost every major biological process. Intriguing features of small RNAs, such as their mechanisms of amplification, rapid evolution and non-cell-autonomous function, bestow upon them the capacity to function as agents of intercellular communications in development, reproduction and immunity, and even in transgenerational inheritance. Although there are many types of extracellular small RNAs, and despite decades of research, the capacity of these molecules to transmit signals between cells and between organisms is still highly controversial. In this Review, we discuss evidence from different plants and animals that small RNAs can act in a non-cell-autonomous manner and even exchange information between species. We also discuss mechanistic insights into small RNA communications, such as the nature of the mobile agents, small RNA signal amplification during transit, signal perception and small RNA activity at the destination.
This is a preview of subscription content, access via your institution
Access options
Access Nature and 54 other Nature Portfolio journals
Get Nature+, our best-value online-access subscription
24,99 € / 30 days
cancel any time
Subscribe to this journal
Receive 12 print issues and online access
176,64 € per year
only 14,72 € per issue
Buy this article
- Purchase on Springer Link
- Instant access to full article PDF
Prices may be subject to local taxes which are calculated during checkout
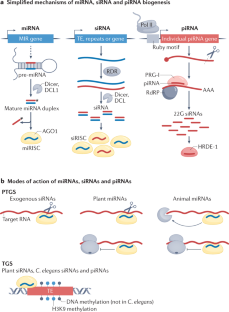
Similar content being viewed by others
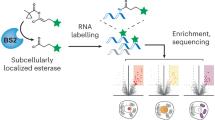
Bioorthogonal masked acylating agents for proximity-dependent RNA labelling
Shubhashree Pani, Tian Qiu, … Bryan C. Dickinson
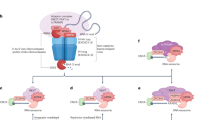
Nuclear mRNA decay: regulatory networks that control gene expression
Xavier Rambout & Lynne E. Maquat
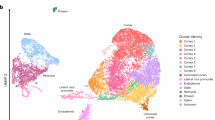
Spatial co-transcriptomics reveals discrete stages of the arbuscular mycorrhizal symbiosis
Karen Serrano, Margaret Bezrutczyk, … Benjamin Cole
Fagard, M. & Vaucheret, H. (Trans)Gene silencing in plants: how many mechanisms? Annu Rev. Plant Physiol. Plant Mol. Biol. 51 , 167–194 (2000).
CAS PubMed Google Scholar
Palauqui, J. C., Elmayan, T., Pollien, J. M. & Vaucheret, H. Systemic acquired silencing: transgene-specific post-transcriptional silencing is transmitted by grafting from silenced stocks to non-silenced scions. EMBO J. 16 , 4738–4745 (1997).
CAS PubMed PubMed Central Google Scholar
Voinnet, O. & Baulcombe, D. C. Systemic signalling in gene silencing. Nature 389 , 553 (1997).
Fire, A. et al. Potent and specific genetic interference by double-stranded RNA in Caenorhabditis elegans. Nature 391 , 806–811 (1998).
Timmons, L. & Fire, A. Specific interference by ingested dsRNA. Nature 395 , 854 (1998).
Baulcombe, D. C. VIGS, HIGS and FIGS: small RNA silencing in the interactions of viruses or filamentous organisms with their plant hosts. Curr. Opin. Plant Biol. 26 , 141–146 (2015).
Koch, A. & Kogel, K. H. New wind in the sails: improving the agronomic value of crop plants through RNAi-mediated gene silencing. Plant Biotechnol. J. 12 , 821–831 (2014).
Petit, J. D., Li, Z. P., Nicolas, W. J., Grison, M. S. & Bayer, E. M. Dare to change, the dynamics behind plasmodesmata-mediated cell-to-cell communication. Curr. Opin. Plant Biol. 53 , 80–89 (2020).
Esau, K. & Thorsch, J. Sieve plate pores and plasmodesmata, the communication channels of the symplast: ultrastructural aspects and developmental relations. Am. J. Bot. 72 , 1641–1653 (1985).
Google Scholar
Ross-Elliott, T. J. et al. Phloem unloading in Arabidopsis roots is convective and regulated by the phloem-pole pericycle. eLife 6 , e24125 (2017).
PubMed PubMed Central Google Scholar
Chen, W. et al. A genetic network for systemic RNA silencing in plants. Plant Physiol. 176 , 2700–2719 (2018).
Melnyk, C. W., Molnar, A., Bassett, A. & Baulcombe, D. C. Mobile 24 nt small RNAs direct transcriptional gene silencing in the root meristems of Arabidopsis thaliana . Curr. Biol. 21 , 1678–1683 (2011).
Molnar, A. et al. Small silencing RNAs in plants are mobile and direct epigenetic modification in recipient cells. Science 328 , 872–875 (2010).
Taochy, C. et al. A genetic screen for impaired systemic RNAi highlights the crucial role of DICER-LIKE 2. Plant Physiol. 175 , 1424–1437 (2017).
Brosnan, C. A. et al. Nuclear gene silencing directs reception of long-distance mRNA silencing in Arabidopsis. Proc. Natl Acad. Sci. USA 104 , 14741–14746 (2007).
Dunoyer, P., Himber, C., Ruiz-Ferrer, V., Alioua, A. & Voinnet, O. Intra- and intercellular RNA interference in Arabidopsis thaliana requires components of the microRNA and heterochromatic silencing pathways. Nat. Genet. 39 , 848–856 (2007).
Schwach, F., Vaistij, F. E., Jones, L. & Baulcombe, D. C. An RNA-dependent RNA polymerase prevents meristem invasion by potato virus X and is required for the activity but not the production of a systemic silencing signal. Plant Physiol. 138 , 1842–1852 (2005).
Smith, L. M. et al. An SNF2 protein associated with nuclear RNA silencing and the spread of a silencing signal between cells in Arabidopsis. Plant Cell 19 , 1507–1521 (2007).
Chen, H. M. et al. 22-nucleotide RNAs trigger secondary siRNA biogenesis in plants. Proc. Natl Acad. Sci. USA 107 , 15269–15274 (2010).
Cuperus, J. T. et al. Unique functionality of 22-nt miRNAs in triggering RDR6-dependent siRNA biogenesis from target transcripts in Arabidopsis. Nat. Struct. Mol. Biol. 17 , 997–1003 (2010).
Mlotshwa, S. et al. DICER-LIKE2 plays a primary role in transitive silencing of transgenes in Arabidopsis. PLoS ONE 3 , e1755 (2008).
Parent, J. S., Bouteiller, N., Elmayan, T. & Vaucheret, H. Respective contributions of Arabidopsis DCL2 and DCL4 to RNA silencing. Plant J. 81 , 223–232 (2015).
Borges, F. & Martienssen, R. A. The expanding world of small RNAs in plants. Nat. Rev. Mol. Cell Biol. 16 , 727–741 (2015).
Martinez, G. et al. Paternal easiRNAs regulate parental genome dosage in Arabidopsis. Nat. Genet. 50 , 193–198 (2018).
Panda, K., McCue, A. D. & Slotkin, R. K. Arabidopsis RNA polymerase IV generates 21-22 nucleotide small RNAs that can participate in RNA-directed DNA methylation and may regulate genes. Phil. Trans. R. Soc. B 375 , 20190417 (2020).
Voinnet, O., Vain, P., Angell, S. & Baulcombe, D. C. Systemic spread of sequence-specific transgene RNA degradation in plants is initiated by localized introduction of ectopic promoterless DNA. Cell 95 , 177–187 (1998).
Devers, E. A. et al. Movement and differential consumption of short interfering RNA duplexes underlie mobile RNA interference. Nat. Plants 6 , 789–799 (2020).
Brosnan, C. A. et al. Genome-scale, single-cell-type resolution of microRNA activities within a whole plant organ. EMBO J. 38 , e100754 (2019).
Su, Z. et al. Regulation of female germline specification via small RNA mobility in Arabidopsis. Plant Cell 32 , 2842–2854 (2020).
Rosas-Diaz, T. et al. A virus-targeted plant receptor-like kinase promotes cell-to-cell spread of RNAi. Proc. Natl Acad. Sci. USA 115 , 1388–1393 (2018).
Tijsterman, M., May, R. C., Simmer, F., Okihara, K. L. & Plasterk, R. H. Genes required for systemic RNA interference in Caenorhabditis elegans . Curr. Biol. 14 , 111–116 (2004).
Timmons, L., Tabara, H., Mello, C. C. & Fire, A. Z. Inducible systemic RNA silencing in Caenorhabditis elegans . Mol. Biol. Cell 14 , 2972–2983 (2003).
Winston, W. M., Sutherlin, M., Wright, A. J., Feinberg, E. H. & Hunter, C. P. Caenorhabditis elegans SID-2 is required for environmental RNA interference. Proc. Natl Acad. Sci. USA 104 , 10565–10570 (2007).
Winston, W. M., Molodowitch, C. & Hunter, C. P. Systemic RNAi in C. elegans requires the putative transmembrane protein SID-1. Science 295 , 2456–2459 (2002).
Jose, A. M., Smith, J. J. & Hunter, C. P. Export of RNA silencing from C. elegans tissues does not require the RNA channel SID-1. Proc. Natl Acad. Sci. USA 106 , 2283–2288 (2009).
Shih, J. D. & Hunter, C. P. SID-1 is a dsRNA-selective dsRNA-gated channel. RNA 17 , 10, 1165-57 (2011).
Marré, J., Traver, E. C. & Jose, A. M. Extracellular RNA is transported from one generation to the next in Caenorhabditis elegans . Proc. Natl Acad. Sci. USA 113 , 12496–12501 (2016).
Whangbo, J. S., Weisman, A. S., Chae, J. & Hunter, C. P. SID-1 domains Important for dsRNA import in Caenorhabditis elegans . G3 7 , 3887–3899 (2017).
Felix, M. A. et al. Natural and experimental infection of Caenorhabditis nematodes by novel viruses related to nodaviruses. PLoS Biol. 9 , e1000586 (2011).
Gammon, D. B. et al. The antiviral RNA interference response provides resistance to lethal Arbovirus infection and vertical transmission in Caenorhabditis elegans . Curr. Biol. 27 , 795–806 (2017).
Rechavi, O., Minevich, G. & Hobert, O. Transgenerational inheritance of an acquired small RNA-based antiviral response in C. elegans . Cell 147 , 1248–1256 (2011).
Zhuang, J. J. & Hunter, C. P. Tissue specificity of Caenorhabditis elegans enhanced RNA interference mutants. Genetics 188 , 235–237 (2011).
McEwan, D. L., Weisman, A. S. & Hunter, C. P. Uptake of extracellular double-stranded RNA by SID-2. Mol. Cell 47 , 746–754 (2012).
Braukmann, F., Jordan, D., Jenkins, B., Koulman, A. & Miska, E. A. SID-2 negatively regulates development likely independent of nutritional dsRNA uptake. RNA Biol. 18 , 888–899 (2021).
Nuez, I. & Felix, M. A. Evolution of susceptibility to ingested double-stranded RNAs in Caenorhabditis nematodes . PLoS ONE 7 , e29811 (2012).
Kaletsky, R. et al. C. elegans interprets bacterial non-coding RNAs to learn pathogenic avoidance. Nature 586 , 445–451 (2020).
Rechavi, O. Transgenerational inheritance: that pathogen gut feeling. Curr. Biol. 30 , R1486–R1488 (2020).
Jose, A. M., Kim, Y. A., Leal-Ekman, S. & Hunter, C. P. Conserved tyrosine kinase promotes the import of silencing RNA into Caenorhabditis elegans cells. Proc. Natl Acad. Sci. USA 109 , 14520–14525 (2012).
Bhatia, S. & Hunter, C. P. SID-4/NCK-1 is important for dsRNA import in Caenorhabditis elegans . Preprint at bioRxiv https://doi.org/10.1101/702019 (2019).
Article Google Scholar
Hinas, A., Wright, A. J. & Hunter, C. P. SID-5 is an endosome-associated protein required for efficient systemic RNAi in C. elegans . Curr. Biol. 22 , 1938–1943 (2012).
Jose, A. M. Movement of regulatory RNA between animal cells. Genesis 53 , 395–416 (2015).
Imae, R., Dejima, K., Kage-Nakadai, E., Arai, H. & Mitani, S. Endomembrane-associated RSD-3 is important for RNAi induced by extracellular silencing RNA in both somatic and germ cells of Caenorhabditis elegans . Sci. Rep. 6 , 28198 (2016).
Wang, E. & Hunter, C. P. SID-1 functions in multiple roles to support parental RNAi in Caenorhabditis elegans . Genetics 207 , 547–557 (2017).
Preston, M. A. et al. Unbiased screen of RNA tailing activities reveals a poly(UG) polymerase. Nat. Methods 16 , 437–445 (2019).
Shukla, A. et al. Poly(UG)-tailed RNAs in genome protection and epigenetic inheritance. Nature 582 , 283–288 (2020).
Dzitoyeva, S., Dimitrijevic, N. & Manev, H. Intra-abdominal injection of double-stranded RNA into anesthetized adult Drosophila triggers RNA interference in the central nervous system. Mol. Psychiatry 6 , 665–670 (2001).
Goto, A., Blandin, S., Royet, J., Reichhart, J. M. & Levashina, E. A. Silencing of Toll pathway components by direct injection of double-stranded RNA into Drosophila adult flies. Nucleic Acids Res. 31 , 6619–6623 (2003).
Petruk, S. et al. Transcription of bxd noncoding RNAs promoted by trithorax represses Ubx in cis by transcriptional interference. Cell 127 , 1209–1221 (2006).
Miller, S. C., Brown, S. J. & Tomoyasu, Y. Larval RNAi in Drosophila? Dev. Genes Evol. 218 , 505–510 (2008).
Tomoyasu, Y. et al. Exploring systemic RNA interference in insects: a genome-wide survey for RNAi genes in Tribolium. Genome Biol. 9 , R10 (2008).
Saleh, M.-C. et al. The endocytic pathway mediates cell entry of dsRNA to induce RNAi silencing. Nat. Cell Biol. 8 , 793–802 (2006).
Ulvila, J. et al. Double-stranded RNA is internalized by scavenger receptor-mediated endocytosis in Drosophila S2 cells. J. Biol. Chem. 281 , 14370–14375 (2006).
Xiao, D. et al. Clathrin-dependent endocytosis plays a predominant role in cellular uptake of double-stranded RNA in the red flour beetle. Insect Biochem. Mol. Biol. 60 , 68–77 (2015).
Vickers, K. C., Palmisano, B. T., Shoucri, B. M., Shamburek, R. D. & Remaley, A. T. MicroRNAs are transported in plasma and delivered to recipient cells by high-density lipoproteins. Nat. Cell Biol. 13 , 423–433 (2011).
Karlikow, M. et al. Drosophila cells use nanotube-like structures to transfer dsRNA and RNAi machinery between cells. Sci. Rep. 6 , 27085 (2016).
Vance, V. & Vaucheret, H. RNA silencing in plants–defense and counterdefense. Science 292 , 2277–2280 (2001).
Gammon, D. B. Caenorhabditis elegans as an emerging model for virus-host interactions. J Virol. 91 , e00509-17 (2017).
Garcia-Ruiz, H. et al. Arabidopsis RNA-dependent RNA polymerases and dicer-like proteins in antiviral defense and small interfering RNA biogenesis during Turnip Mosaic Virus infection. Plant Cell 22 , 481–496 (2010).
Wang, X. B. et al. RNAi-mediated viral immunity requires amplification of virus-derived siRNAs in Arabidopsis thaliana . Proc. Natl Acad. Sci. USA 107 , 484–489 (2010).
Saleh, M.-C. et al. Antiviral immunity in Drosophila requires systemic RNA interference spread. Nature 458 , 346–350 (2009).
Tassetto, M., Kunitomi, M. & Andino, R. Circulating immune cells mediate a systemic RNAi-based adaptive antiviral response in Drosophila . Cell 169 , 314–325.e13 (2017).
Goic, B. et al. RNA-mediated interference and reverse transcription control the persistence of RNA viruses in the insect model Drosophila. Nat. Immunol. 14 , 396–403 (2013).
Goic, B. et al. Virus-derived DNA drives mosquito vector tolerance to arboviral infection. Nat. Commun. 7 , 12410 (2016).
Poirier, E. Z. et al. Dicer-2-dependent generation of viral DNA from defective genomes of RNA viruses modulates antiviral immunity in insects. Cell Host Microbe 23 , 353–365.e8 (2018).
Suzuki, Y. et al. Non-retroviral endogenous viral element limits cognate virus replication in Aedes aegypti ovaries. Curr. Biol. 30 , 3495–3506.e6 (2020).
Maori, E. et al. IAPV, a bee-affecting virus associated with colony collapse disorder can be silenced by dsRNA ingestion. Insect Mol. Biol. 18 , 55–60 (2009).
Maori, E. et al. A secreted RNA binding protein forms RNA-stabilizing granules in the honeybee royal jelly. Mol. Cell 74 , 598–608.e6 (2019).
Maori, E. et al. A transmissible RNA pathway in honey bees. Cell Rep. 27 , 1949–1959.e6 (2019).
Houri-Zeevi, L. & Rechavi, O. A matter of time: small RNAs regulate the duration of epigenetic inheritance. Trends Genet. 33 , 46–57 (2017).
Zhuang, J. J. & Hunter, C. P. The influence of competition among C. elegans small RNA pathways on development. Genes 3 , 671–685 (2012).
Sarkies, P., Ashe, A., Le Pen, J., McKie, M. A. & Miska, E. A. Competition between virus-derived and endogenous small RNAs regulates gene expression in Caenorhabditis elegans. Genome Res. 23 , 1258–1270 (2013).
You, C. et al. FIERY1 promotes microRNA accumulation by suppressing rRNA-derived small interfering RNAs in Arabidopsis. Nat. Commun. 10 , 4424 (2019).
Wahba, L., Hansen, L. & Fire, A. Z. An essential role for the piRNA pathway in regulating the ribosomal RNA pool in C. elegans . Dev. Cell 56 , 2295–2312.e6 (2021).
Li, S. et al. Unidirectional movement of small RNAs from shoots to roots in interspecific heterografts. Nat. Plants 7 , 50–59 (2021).
Leslie, M. NIH effort gambles on mysterious extracellular RNAs. Science 341 , 947 (2013).
Posner, R. et al. Neuronal small RNAs control behavior transgenerationally. Cell 177 , 1814–1826.e15 (2019).
Liu, Y., Teng, C., Xia, R. & Meyers, B. C. PhasiRNAs in plants: their biogenesis, genic sources, and roles in stress responses, development, and reproduction. Plant Cell 32 , 3059–3080 (2020).
Garcia, D., Collier, S. A., Byrne, M. E. & Martienssen, R. A. Specification of leaf polarity in Arabidopsis via the trans-acting siRNA pathway. Curr. Biol. 16 , 933–938 (2006).
Nogueira, F. T., Madi, S., Chitwood, D. H., Juarez, M. T. & Timmermans, M. C. Two small regulatory RNAs establish opposing fates of a developmental axis. Genes Dev. 21 , 750–755 (2007).
Xu, L. et al. Genetic interaction between the AS1-AS2 and RDR6-SGS3-AGO7 pathways for leaf morphogenesis. Plant Cell Physiol. 47 , 853–863 (2006).
Adenot, X. et al. DRB4-dependent TAS3 trans-acting siRNAs control leaf morphology through AGO7. Curr. Biol. 16 , 927–932 (2006).
Fahlgren, N. et al. Regulation of AUXIN RESPONSE FACTOR3 by TAS3 ta-siRNA affects developmental timing and patterning in Arabidopsis. Curr. Biol. 16 , 939–944 (2006).
Hunter, C. et al. Trans-acting siRNA-mediated repression of ETTIN and ARF4 regulates heteroblasty in Arabidopsis. Development 133 , 2973–2981 (2006).
Peragine, A., Yoshikawa, M., Wu, G., Albrecht, H. L. & Poethig, R. S. SGS3 and SGS2 / SDE1 / RDR6 are required for juvenile development and the production of trans-acting siRNAs in Arabidopsis. Genes. Dev. 18 , 2368–2379 (2004).
Yoshikawa, M., Peragine, A., Park, M. Y. & Poethig, R. S. A pathway for the biogenesis of trans-acting siRNAs in Arabidopsis. Genes. Dev. 19 , 2164–2175 (2005).
Hobecker, K. V. et al. The microRNA390/ TAS3 pathway mediates symbiotic nodulation and lateral root growth. Plant Physiol. 174 , 2469–2486 (2017).
Marin, E. et al. miR390, Arabidopsis TAS3 tasiRNAs, and their AUXIN RESPONSE FACTOR targets define an autoregulatory network quantitatively regulating lateral root growth. Plant Cell 22 , 1104–1117 (2010).
Yoon, E. K. et al. Auxin regulation of the microRNA390-dependent transacting small interfering RNA pathway in Arabidopsis lateral root development. Nucleic Acids Res. 38 , 1382–1391 (2010).
Li, X. et al. The REL3 -mediated TAS3 ta-siRNA pathway integrates auxin and ethylene signaling to regulate nodulation in Lotus japonicus . N. Phytol. 201 , 531–544 (2014).
CAS Google Scholar
Nagasaki, H. et al. The small interfering RNA production pathway is required for shoot meristem initiation in rice. Proc. Natl Acad. Sci. USA 104 , 14867–14871 (2007).
Su, Z. et al. The THO complex non-cell-autonomously represses female germline specification through the TAS3-ARF3 module. Curr. Biol. 27 , 1597–1609.e2 (2017).
Ding, B. et al. Developmental genetics of corolla tube formation: role of the tasiRNA-ARF pathway and a conceptual model. Plant Cell 32 , 3452–3468 (2020).
Chitwood, D. H. et al. Pattern formation via small RNA mobility. Genes Dev. 23 , 549–554 (2009).
Johnson, C. et al. Clusters and superclusters of phased small RNAs in the developing inflorescence of rice. Genome Res. 19 , 1429–1440 (2009).
Yang, X. et al. Widespread occurrence of microRNA-mediated target cleavage on membrane-bound polysomes. Genome Biol. 22 , 15 (2021).
Zhai, J. et al. Spatiotemporally dynamic, cell-type-dependent premeiotic and meiotic phasiRNAs in maize anthers. Proc. Natl Acad. Sci. USA 112 , 3146–3151 (2015).
Araki, S. et al. miR2118-dependent U-rich phasiRNA production in rice anther wall development. Nat. Commun. 11 , 3115 (2020).
Fan, Y. et al. PMS1T, producing phased small-interfering RNAs, regulates photoperiod-sensitive male sterility in rice. Proc. Natl Acad. Sci. USA 113 , 15144–15149 (2016).
Tamim, S. et al. Cis-directed cleavage and nonstoichiometric abundances of 21-nucleotide reproductive phased small interfering RNAs in grasses. N. Phytol. 220 , 865–877 (2018).
Zhou, H. et al. Photoperiod- and thermo-sensitive genic male sterility in rice are caused by a point mutation in a novel noncoding RNA that produces a small RNA. Cell Res. 22 , 649–660 (2012).
Komiya, R. et al. Rice germline-specific Argonaute MEL1 protein binds to phasiRNAs generated from more than 700 lincRNAs. Plant J. 78 , 385–397 (2014).
Teng, C. et al. Dicer-like 5 deficiency confers temperature-sensitive male sterility in maize. Nat. Commun. 11 , 2912 (2020).
Jiang, P. et al. 21-nt phasiRNAs direct target mRNA cleavage in rice male germ cells. Nat. Commun. 11 , 5191 (2020).
Tian, P. et al. Evolution and diversification of reproductive phased small interfering RNAs in Oryza species. N. Phytol. 229 , 2970–2983 (2021).
Zhang, Y. C. et al. Reproductive phasiRNAs regulate reprogramming of gene expression and meiotic progression in rice. Nat. Commun. 11 , 6031 (2020).
Kasschau, K. D. et al. Genome-wide profiling and analysis of Arabidopsis siRNAs. PLoS Biol. 5 , e57 (2007).
Long, J. et al. Nurse cell–derived small RNAs define paternal epigenetic inheritance in Arabidopsis. Science 373 , eabh0556 (2021).
Slotkin, R. K. et al. Epigenetic reprogramming and small RNA silencing of transposable elements in pollen. Cell 136 , 461–472 (2009).
Martinez, G., Panda, K., Kohler, C. & Slotkin, R. K. Silencing in sperm cells is directed by RNA movement from the surrounding nurse cell. Nat. Plants 2 , 16030 (2016).
Klesen, S., Hill, K. & Timmermans, M. C. P. Small RNAs as plant morphogens. Curr. Top. Dev. Biol. 137 , 455–480 (2020).
Yao, X. et al. Two types of cis-acting elements control the abaxial epidermis-specific transcription of the MIR165a and MIR166a genes. FEBS Lett. 583 , 3711–3717 (2009).
Emery, J. F. et al. Radial patterning of Arabidopsis shoots by class III HD-ZIP and KANADI genes. Curr. Biol. 13 , 1768–1774 (2003).
Juarez, M. T., Kui, J. S., Thomas, J., Heller, B. A. & Timmermans, M. C. microRNA-mediated repression of rolled leaf1 specifies maize leaf polarity. Nature 428 , 84–88 (2004).
McConnell, J. R. et al. Role of PHABULOSA and PHAVOLUTA in determining radial patterning in shoots. Nature 411 , 709–713 (2001).
Carlsbecker, A. et al. Cell signalling by microRNA165/6 directs gene dose-dependent root cell fate. Nature 465 , 316–321 (2010).
Miyashima, S., Koi, S., Hashimoto, T. & Nakajima, K. Non-cell-autonomous microRNA165 acts in a dose-dependent manner to regulate multiple differentiation status in the Arabidopsis root. Development 138 , 2303–2313 (2011).
Knauer, S. et al. A protodermal miR394 signal defines a region of stem cell competence in the Arabidopsis shoot meristem. Dev. Cell 24 , 125–132 (2013).
Buhtz, A., Springer, F., Chappell, L., Baulcombe, D. C. & Kehr, J. Identification and characterization of small RNAs from the phloem of Brassica napus . Plant J. 53 , 739–749 (2008).
Yoo, B. C. et al. A systemic small RNA signaling system in plants. Plant Cell 16 , 1979–2000 (2004).
Lin, S. I. et al. Regulatory network of microRNA399 and PHO2 by systemic signaling. Plant Physiol. 147 , 732–746 (2008).
Buhtz, A., Pieritz, J., Springer, F. & Kehr, J. Phloem small RNAs, nutrient stress responses, and systemic mobility. BMC Plant Biol. 10 , 64 (2010).
Okuma, N., Soyano, T., Suzaki, T. & Kawaguchi, M. MIR2111-5 locus and shoot-accumulated mature miR2111 systemically enhance nodulation depending on HAR1 in Lotus japonicus . Nat. Commun. 11 , 5192 (2020).
Tsikou, D. et al. Systemic control of legume susceptibility to rhizobial infection by a mobile microRNA. Science 362 , 233–236 (2018).
Skopelitis, D. S., Benkovics, A. H., Husbands, A. Y. & Timmermans, M. C. P. Boundary formation through a direct threshold-based readout of mobile small RNA gradients. Dev. Cell 43 , 265–273.e6 (2017).
Vaten, A. et al. Callose biosynthesis regulates symplastic trafficking during root development. Dev. Cell 21 , 1144–1155 (2011).
Skopelitis, D. S. et al. Gating of miRNA movement at defined cell-cell interfaces governs their impact as positional signals. Nat. Commun. 9 , 3107 (2018).
Liu, Q. et al. The ARGONAUTE10 gene modulates shoot apical meristem maintenance and establishment of leaf polarity by repressing miR165/166 in Arabidopsis. Plant J. 58 , 27–40 (2009).
Zhu, H. et al. Arabidopsis Argonaute10 specifically sequesters miR166/165 to regulate shoot apical meristem development. Cell 145 , 242–256 (2011).
Cai, H. et al. Spatiotemporal control of miR398 biogenesis via chromatin remodeling and kinase signaling ensures proper ovule development. Plant Cell 33 , 1530–1553 (2021).
de Felippes, F. F., Ott, F. & Weigel, D. Comparative analysis of non-autonomous effects of tasiRNAs and miRNAs in Arabidopsis thaliana . Nucleic Acids Res. 39 , 2880–2889 (2011).
PubMed Google Scholar
Bologna, N. G. et al. Nucleo-cytosolic shuttling of ARGONAUTE1 prompts a revised model of the plant microRNA pathway. Mol. Cell 69 , 709–719.e5 (2018).
Li, S. et al. Biogenesis of phased siRNAs on membrane-bound polysomes in Arabidopsis . eL ife 5 , e22750 (2016).
Jouannet, V. et al. Cytoplasmic Arabidopsis AGO7 accumulates in membrane-associated siRNA bodies and is required for ta-siRNA biogenesis. EMBO J. 31 , 1704–1713 (2012).
Dalmadi, A., Gyula, P., Balint, J., Szittya, G. & Havelda, Z. AGO-unbound cytosolic pool of mature miRNAs in plant cells reveals a novel regulatory step at AGO1 loading. Nucleic Acids Res. 47 , 9803–9817 (2019).
Ow, M. C., Borziak, K., Nichitean, A. M., Dorus, S. & Hall, S. E. Early experiences mediate distinct adult gene expression and reproductive programs in Caenorhabditis elegans. PLOS Genet. 14 , e1007219 (2018).
Ohno, H. & Bao, Z. Small RNAs couple embryonic developmental programs to gut microbes. Preprint at bioRxiv https://doi.org/10.1101/2020.11.13.381830 (2020).
Long, O. S. et al. A C. elegans model of human α1-antitrypsin deficiency links components of the RNAi pathway to misfolded protein turnover. Hum. Mol. Genet. 23 , 5109–5122 (2014).
Setten, R. L., Rossi, J. J. & Han, S.-P. The current state and future directions of RNAi-based therapeutics. Nat. Rev. Drug Discov. 18 , 421–446 (2019).
Valadi, H. et al. Exosome-mediated transfer of mRNAs and microRNAs is a novel mechanism of genetic exchange between cells. Nat. Cell Biol. 9 , 654–659 (2007).
Shurtleff, M. J., Temoche-Diaz, M. M., Karfilis, K. V., Ri, S. & Schekman, R. Y-box protein 1 is required to sort microRNAs into exosomes in cells and in a cell-free reaction. eLife 5 , e19276 (2016).
O’Brien, K., Breyne, K., Ughetto, S., Laurent, L. C. & Breakefield, X. O. RNA delivery by extracellular vesicles in mammalian cells and its applications. Nat. Rev. Mol. Cell Biol. 21 , 585–606 (2020).
Thomou, T. et al. Adipose-derived circulating miRNAs regulate gene expression in other tissues. Nature 542 , 450–455 (2017).
Witwer, K. W. et al. Standardization of sample collection, isolation and analysis methods in extracellular vesicle research. J. Extracell. Vesicles 2 , 20360 (2013).
Kim, Y.-K., Yeo, J., Kim, B., Ha, M. & Kim, V. N. Short structured RNAs with low GC content are selectively lost during extraction from a small number of cells. Mol. Cell 46 , 893–895 (2012).
Ostrowski, M. et al. Rab27a and Rab27b control different steps of the exosome secretion pathway. Nat. Cell Biol. 12 , 19–30 (2010).
Chevillet, J. R. et al. Quantitative and stoichiometric analysis of the microRNA content of exosomes. Proc. Natl Acad. Sci. USA 111 , 14888–14893 (2014).
Das, S. et al. The Extracellular RNA Communication Consortium: establishing foundational knowledge and technologies for extracellular RNA research. Cell 177 , 231–242 (2019).
Srinivasan, S. et al. Small RNA sequencing across diverse biofluids identifies optimal methods for exRNA isolation. Cell 177 , 446–462.e16 (2019).
Zhang, H. et al. Identification of distinct nanoparticles and subsets of extracellular vesicles by asymmetric flow field-flow fractionation. Nat. Cell Biol. 20 , 332–343 (2018).
Shurtleff, M. J. et al. Broad role for YBX1 in defining the small noncoding RNA composition of exosomes. Proc. Natl Acad. Sci. USA 114 , E8987–E8995 (2017).
Villarroya-Beltri, C. et al. Sumoylated hnRNPA2B1 controls the sorting of miRNAs into exosomes through binding to specific motifs. Nat. Commun. 4 , 2980 (2013).
Bolukbasi, M. F. et al. miR-1289 and “zipcode”-like sequence enrich mRNAs in microvesicles. Mol. Ther. Nucleic Acids 1 , e10 (2012).
Koppers-Lalic, D. et al. Nontemplated nucleotide additions distinguish the small RNA composition in cells from exosomes. Cell Rep. 8 , 1649–1658 (2014).
Clancy, J. W., Zhang, Y., Sheehan, C. & D’Souza-Schorey, C. An ARF6–exportin-5 axis delivers pre-miRNA cargo to tumour microvesicles. Nat. Cell Biol. 21 , 856–866 (2019).
Leidal, A. M. et al. The LC3-conjugation machinery specifies the loading of RNA-binding proteins into extracellular vesicles. Nat. Cell Biol. 22 , 187–199 (2020).
McKenzie, A. J. et al. KRAS-MEK signaling controls Ago2 sorting into exosomes. Cell Rep. 15 , 978–987 (2016).
Matsuzaki, J. & Ochiya, T. Extracellular microRNAs and oxidative stress in liver injury: a systematic mini review. J. Clin. Biochem. Nutr. 63 , 6–11 (2018).
Beninson, L. A. et al. Acute stressor exposure modifies plasma exosome-associated heat shock protein 72 (Hsp72) and microRNA (miR-142-5p and miR-203). PLoS ONE 9 , e108748 (2014).
Ma, C. et al. Moderate exercise enhances endothelial progenitor cell exosomes release and function. Med. Sci. Sports Exerc. 50 , 2024–2032 (2018).
Arroyo, J. D. et al. Argonaute2 complexes carry a population of circulating microRNAs independent of vesicles in human plasma. Proc. Natl Acad. Sci. USA 108 , 5003–5008 (2011).
Turchinovich, A., Weiz, L., Langheinz, A. & Burwinkel, B. Characterization of extracellular circulating microRNA. Nucleic Acids Res. 39 , 7223–7233 (2011).
Wang, K., Zhang, S., Weber, J., Baxter, D. & Galas, D. J. Export of microRNAs and microRNA-protective protein by mammalian cells. Nucleic Acids Res. 38 , 7248–7259 (2010).
Rechavi, O. et al. Trans-SILAC: sorting out the non-cell-autonomous proteome. Nat. Methods 7 , 923–927 (2010).
Rechavi, O. et al. Cell contact-dependent acquisition of cellular and viral nonautonomously encoded small RNAs. Genes Dev. 23 , 1971–1979 (2009).
Valiunas, V. et al. Connexin-specific cell-to-cell transfer of short interfering RNA by gap junctions: oligonucleotide and siRNA transfer through gap junctions. J. Physiol. 568 , 459–468 (2005).
Kizana, E., Cingolani, E. & Marbán, E. Non-cell-autonomous effects of vector-expressed regulatory RNAs in mammalian heart cells. Gene Ther. 16 , 1163–1168 (2009).
Katakowski, M., Buller, B., Wang, X., Rogers, T. & Chopp, M. Functional microRNA is transferred between glioma cells. Cancer Res. 70 , 8259–8263 (2010).
Lim, P. K. et al. Gap junction-mediated import of microRNA from bone marrow stromal cells can elicit cell cycle quiescence in breast cancer cells. Cancer Res. 71 , 1550–1560 (2011).
Lu, J. J., Yang, W. M., Li, F., Zhu, W. & Chen, Z. Tunneling nanotubes mediated microRNA-155 intercellular transportation promotes bladder cancer cells’ invasive and proliferative capacity. Int. J. Nanomed. 14 , 9731–9743 (2019).
Morales, C. R. et al. A TB-RBP and Ter ATPase complex accompanies specific mRNAs from nuclei through the nuclear pores and into intercellular bridges in mouse male germ cells. Dev. Biol. 246 , 480–494 (2002).
Bermúdez-Barrientos, J. R., Ramírez-Sánchez, O., Chow, F. W.-N., Buck, A. H. & Abreu-Goodger, C. Disentangling sRNA-Seq data to study RNA communication between species. Nucleic Acids Res. 48 , e21 (2020).
Shahid, S. et al. MicroRNAs from the parasitic plant Cuscuta campestris target host messenger RNAs. Nature 553 , 82–85 (2018).
Zhang, T. et al. Cotton plants export microRNAs to inhibit virulence gene expression in a fungal pathogen. Nat. Plants 2 , 16153 (2016).
Cai, Q. et al. Plants send small RNAs in extracellular vesicles to fungal pathogen to silence virulence genes. Science 360 , 1126–1129 (2018).
Dunker, F. et al. Oomycete small RNAs bind to the plant RNA-induced silencing complex for virulence. eLife 9 , e56096 (2020).
Hou, Y. et al. A Phytophthora effector suppresses trans-kingdom RNAi to promote disease susceptibility. Cell Host Microbe 25 , 153–165.e5 (2019).
Ren, B., Wang, X., Duan, J. & Ma, J. Rhizobial tRNA-derived small RNAs are signal molecules regulating plant nodulation. Science 365 , 919–922 (2019).
Wang, B. et al. Puccinia striiformis f. sp. tritici microRNA-like RNA 1 (Pst-milR1), an important pathogenicity factor of Pst, impairs wheat resistance to Pst by suppressing the wheat pathogenesis-related 2 gene. N. Phytol. 215 , 338–350 (2017).
Weiberg, A. et al. Fungal small RNAs suppress plant immunity by hijacking host RNA interference pathways. Science 342 , 118–123 (2013).
Qiao, Y. et al. Oomycete pathogens encode RNA silencing suppressors. Nat. Genet. 45 , 330–333 (2013).
Rutter, B. D. & Innes, R. W. Extracellular vesicles isolated from the leaf apoplast carry stress-response proteins. Plant Physiol. 173 , 728–741 (2017).
Baldrich, P. et al. Plant extracellular vesicles contain diverse small RNA species and are enriched in 10- to 17-nucleotide “tiny” RNAs. Plant Cell 31 , 315–324 (2019).
He, B. et al. RNA-binding proteins contribute to small RNA loading in plant extracellular vesicles. Nat. Plants 7 , 342–352 (2021).
Rutter, B. D. & Innes, R. W. Growing pains: addressing the pitfalls of plant extracellular vesicle research. N. Phytol. 228 , 1505–1510 (2020).
Park, M. S., Sim, G., Kehling, A. C. & Nakanishi, K. Human Argonaute2 and Argonaute3 are catalytically activated by different lengths of guide RNA. Proc. Natl Acad. Sci. USA 117 , 28576–28578 (2020).
Maule, A. G. et al. An eye on RNAi in nematode parasites. Trends Parasitol. 27 , 505–513 (2011).
Liu, H. et al. Escherichia coli noncoding RNAs can affect gene expression and physiology of Caenorhabditis elegans. Nat. Commun. 3 , 1073 (2012).
Akay, A., Sarkies, P. & Miska, E. A. E. coli OxyS non-coding RNA does not trigger RNAi in C. elegans . Sci. Rep. 5 , 9597 (2015).
Buck, A. H. et al. Exosomes secreted by nematode parasites transfer small RNAs to mammalian cells and modulate innate immunity. Nat. Commun. 5 , 5488 (2014).
Quintana, J. F. et al. Comparative analysis of small RNAs released by the filarial nematode Litomosoides sigmodontis in vitro and in vivo. PLoS Neg. Trop. Dis. 13 , e0007811 (2019).
Quintana, J. F. et al. Extracellular Onchocerca-derived small RNAs in host nodules and blood. Parasites Vectors 8 , 58 (2015).
Nowacki, F. C. et al. Protein and small non-coding RNA-enriched extracellular vesicles are released by the pathogenic blood fluke Schistosoma mansoni . J. Extracell. Vesicles 4 , 28665 (2015).
Chow, F. W.-N. et al. Secretion of an Argonaute protein by a parasitic nematode and the evolution of its siRNA guides. Nucleic Acids Res. 47 , 3594–3606 (2019).
Sarkies, P. & Miska, E. A. Molecular biology. Is there social RNA? Science 341 , 467–468 (2013).
Mayoral, J. G. et al. Wolbachia small noncoding RNAs and their role in cross-kingdom communications. Proc. Natl Acad. Sci. USA 111 , 18721–18726 (2014).
Cui, C. et al. A fungal pathogen deploys a small silencing RNA that attenuates mosquito immunity and facilitates infection. Nat. Commun. 10 , 4298 (2019).
Baum, J. A. et al. Control of coleopteran insect pests through RNA interference. Nat. Biotechnol. 25 , 1322–1326 (2007).
Bolognesi, R. et al. Characterizing the mechanism of action of double-stranded RNA activity against western corn rootworm (Diabrotica virgifera virgifera LeConte). PLoS ONE 7 , e47534 (2012).
Miyata, K. et al. Establishing an in vivo assay system to identify components involved in environmental RNA interference in the western corn rootworm. PLoS ONE 9 , e101661 (2014).
Chow, Y. S. et al. in Encyclopedia of Entomology (ed. Capinera, J. L.) 4205–4207 (Springer, 2008).
Zhu, K. et al. Plant microRNAs in larval food regulate honeybee caste development. PLoS Genet. 13 , e1006946 (2017).
Garbian, Y., Maori, E., Kalev, H., Shafir, S. & Sela, I. Bidirectional transfer of RNAi between honey bee and Varroa destructor: Varroa gene silencing reduces Varroa population. PLoS Pathog. 8 , e1003035 (2012).
LaMonte, G. et al. Translocation of sickle cell erythrocyte microRNAs into Plasmodium falciparum inhibits parasite translation and contributes to malaria resistance. Cell Host Microbe 12 , 187–199 (2012).
Wang, Z. et al. Red blood cells release microparticles containing human argonaute 2 and miRNAs to target genes of Plasmodium falciparum : MPs and hAgo2-miRNAs targeting P. falciparum . Emerg. Microbes Infect. 6 , 1–11 (2017).
Liu, S. et al. The host shapes the gut microbiota via fecal microRNA. Cell Host Microbe 19 , 32–43 (2016).
Zhang, L. et al. Exogenous plant MIR168a specifically targets mammalian LDLRAP1: evidence of cross-kingdom regulation by microRNA. Cell Res. 22 , 107–126 (2012).
Chen, Q. et al. SIDT1-dependent absorption in the stomach mediates host uptake of dietary and orally administered microRNAs. Cell Res. 31 , 247–258 (2021).
Tian, Y., Simanshu, D. K., Ma, J.-B. & Patel, D. J. Structural basis for piRNA 2’-O-methylated 3’-end recognition by Piwi PAZ (Piwi/Argonaute/Zwille) domains. Proc. Natl Acad. Sci. USA 108 , 903–910 (2011).
Svoboda, P. in Introduction to RNAi and miRNA pathways 1st edn 313–328 (Karolinum, 2020).
Dickinson, B. et al. Lack of detectable oral bioavailability of plant microRNAs after feeding in mice. Nat. Biotechnol. 31 , 965–967 (2013).
Moriano-Gutierrez, S. et al. The noncoding small RNA SsrA is released by Vibrio fischeri and modulates critical host responses. PLoS Biol. 18 , e3000934 (2020).
Tavora, B. et al. Tumoural activation of TLR3–SLIT2 axis in endothelium drives metastasis. Nature 586 , 299–304 (2020).
Ruby, J. G. et al. Large-scale sequencing reveals 21U-RNAs and additional microRNAs and endogenous siRNAs in C. elegans . Cell 127 , 1193–1207 (2006).
Carthew, R. W. & Sontheimer, E. J. Origins and mechanisms of miRNAs and siRNAs. Cell 136 , 642–655 (2009).
Kobayashi, H. & Tomari, Y. RISC assembly: coordination between small RNAs and Argonaute proteins. Biochim. Biophys. Acta 1859 , 71–81 (2016).
Swarts, D. C. et al. The evolutionary journey of Argonaute proteins. Nat. Struct. Mol. Biol. 21 , 743–753 (2014).
Fang, X. & Qi, Y. RNAi in plants: an Argonaute-centered view. Plant Cell 28 , 272–285 (2016).
Czech, B. et al. piRNA-guided genome defense: from biogenesis to silencing. Annu. Rev. Genet. 52 , 131–157 (2018).
Rajagopalan, R., Vaucheret, H., Trejo, J. & Bartel, D. P. A diverse and evolutionarily fluid set of microRNAs in Arabidopsis thaliana . Genes Dev. 20 , 3407–3425 (2006).
Axtell, M. J. & Meyers, B. C. Revisiting criteria for plant microRNA annotation in the era of big data. Plant Cell 30 , 272–284 (2018).
Kim, V. N. MicroRNA biogenesis: coordinated cropping and dicing. Nat. Rev. Mol. Cell Biol. 6 , 376–385 (2005).
Meyers, B. C. et al. Criteria for annotation of plant microRNAs. Plant Cell 20 , 3186–3190 (2008).
Chapman, E. J. & Carrington, J. C. Specialization and evolution of endogenous small RNA pathways. Nat. Rev. Genet. 8 , 884–896 (2007).
Fukudome, A. & Fukuhara, T. Plant dicer-like proteins: double-stranded RNA-cleaving enzymes for small RNA biogenesis. J. Plant Res. 130 , 33–44 (2017).
Castel, S. E. & Martienssen, R. A. RNA interference in the nucleus: roles for small RNAs in transcription, epigenetics and beyond. Nat. Rev. Genet. 14 , 100–112 (2013).
Ghildiyal, M. & Zamore, P. D. Small silencing RNAs: an expanding universe. Nat. Rev. Genet. 10 , 94–108 (2009).
Gu, S. G. et al. Amplification of siRNA in Caenorhabditis elegans generates a transgenerational sequence-targeted histone H3 lysine 9 methylation footprint. Nat. Genet. 44 , 157–164 (2012).
Mao, H. et al. The Nrde pathway mediates small-RNA-directed histone H3 lysine 27 trimethylation in Caenorhabditis elegans . Curr. Biol. 25 , 2398–2403 (2015).
Schwartz-Orbach, L. et al. Caenorhabditis elegans nuclear RNAi factor SET-32 deposits the transgenerational histone modification, H3K23me3. eLife 9 , e54309 (2020).
Liu, C. et al. Arabidopsis ARGONAUTE 1 binds chromatin to promote gene transcription in response to hormones and stresses. Dev. Cell 44 , 348–361.e7 (2018).
Correa, R. L., Steiner, F. A., Berezikov, E. & Ketting, R. F. MicroRNA-directed siRNA biogenesis in Caenorhabditis elegans . PLoS Genet. 6 , e1000903 (2010).
Tang, W. et al. A sex chromosome piRNA promotes robust dosage compensation and sex determination in C. elegans . Dev. Cell 44 , 762–770.e3 (2018).
Matzke, M. A. & Mosher, R. A. RNA-directed DNA methylation: an epigenetic pathway of increasing complexity. Nat. Rev. Genet. 15 , 394–408 (2014).
Cao, M. et al. Virus infection triggers widespread silencing of host genes by a distinct class of endogenous siRNAs in Arabidopsis . Proc. Natl Acad. Sci. USA 111 , 14613–14618 (2014).
Liu, L. & Chen, X. RNA quality control as a key to suppressing RNA silencing of endogenous genes in plants. Mol. Plant. 9 , 826–836 (2016).
Almeida, M. V., Andrade-Navarro, M. A. & Ketting, R. F. Function and evolution of nematode RNAi pathways. Noncoding RNA 5 , 8 (2019).
CAS PubMed Central Google Scholar
Zhou, X. et al. RdRP-synthesized antisense ribosomal siRNAs silence pre-rRNA via the nuclear RNAi pathway. Nat. Struct. Mol. Biol. 24 , 258–269 (2017).
Ketting, R. F. & Cochella, L. Concepts and functions of small RNA pathways in C. elegans . Curr. Top. Dev. Biol. 144 , 45–89 (2021).
Lev, I. et al. Germ granules govern small RNA inheritance. Curr. Biol. 29 , 2880–2891.e4 (2019).
Rechavi, O. & Lev, I. Principles of transgenerational small RNA inheritance in Caenorhabditis elegans . Curr. Biol. 27 , R720–R730 (2017).
Sapetschnig, A., Sarkies, P., Lehrbach, N. J. & Miska, E. A. Tertiary siRNAs mediate paramutation in C. elegans . PLoS Genet. 11 , e1005078 (2015).
Ashe, A. et al. PiRNAs can trigger a multigenerational epigenetic memory in the germline of C. elegans. Cell 150 , 88–99 (2012).
Buckley, B. A. et al. A nuclear Argonaute promotes multigenerational epigenetic inheritance and germline immortality. Nature 489 , 447–451 (2012).
Luteijn, M. J. et al. Extremely stable Piwi-induced gene silencing in Caenorhabditis elegans : extremely stable Piwi-induced gene silencing. EMBO J. 31 , 3422–3430 (2012).
Shirayama, M. et al. piRNAs initiate an epigenetic memory of nonself RNA in the C. elegans germline. Cell 150 , 65–77 (2012).
Wan, G. et al. Spatiotemporal regulation of liquid-like condensates in epigenetic inheritance. Nature 557 , 679–683 (2018).
Xu, F. et al. A cytoplasmic argonaute protein promotes the inheritance of RNAi. Cell Rep. 23 , 2482–2494 (2018).
Download references
Acknowledgements
The authors are grateful to N. Alon for help with the illustrations. Research on small RNAs in the Chen laboratory is funded by the US National Institutes of Health (GM129373). The Rechavi laboratory is funded by ERC grant no. 819151.
Author information
Authors and affiliations.
Department of Botany and Plant Sciences, Institute for Integrative Genome Biology, University of California, Riverside, CA, USA
Xuemei Chen
Department of Neurobiology, The George S. Wise Faculty of Life Sciences, Tel Aviv University, Tel Aviv, Israel
Oded Rechavi
Sagol School of Neuroscience, Tel Aviv University, Tel Aviv, Israel
You can also search for this author in PubMed Google Scholar
Contributions
The authors contributed equally to all aspects of the article.
Corresponding authors
Correspondence to Xuemei Chen or Oded Rechavi .
Ethics declarations
Competing interests.
The authors declare no competing interests.
Additional information
Peer review information.
Nature Reviews Molecular Cell Biology thanks Shouhong Guang and the other, anonymous, reviewer(s) for their contribution to the peer review of this work.
Publisher’s note
Springer Nature remains neutral with regard to jurisdictional claims in published maps and institutional affiliations.
Silencing of genetic elements usually through RNA degradation or the inhibition of their translation.
RNA-mediated gene silencing in which the trigger for silencing is delivered to, or originates from, cells that are different from those that undergo gene silencing.
The ‘shared’ cytoplasm of nearly all plant cells due to the presence of the plasmodesmata connecting adjacent plant cells.
The extracellular (outside the plasma membrane) environment consisting of the cell wall and other molecules therein.
A tissue in the plant vasculature that transmits nutrients and other molecules to distant plant parts.
Also miRNA–miRNA* duplexes, products of RNA processing by Dicer, consisting of a duplex of small RNAs with an overhang on each strand. The strand that is not loaded into an Argonaute protein is marked with an asterisk.
In Arabidopsis thaliana , cytoplasmic foci containing factors involved in the production of endogenous siRNAs, such as ARGONAUTE 7 (AGO7), SUPPRESSOR OF GENE SILENCING 3 (SGS3) and RNA-DEPENDENT RNA POLYMERASE 6 (RDR6).
The fluid in the plant apoplasm that can be collected through centrifugation.
Silencing of genetic elements at the transcriptional level, usually through the deposition of repressive histone modifications or DNA methylation.
Rights and permissions
Reprints and permissions
About this article
Cite this article.
Chen, X., Rechavi, O. Plant and animal small RNA communications between cells and organisms. Nat Rev Mol Cell Biol 23 , 185–203 (2022). https://doi.org/10.1038/s41580-021-00425-y
Download citation
Accepted : 24 September 2021
Published : 27 October 2021
Issue Date : March 2022
DOI : https://doi.org/10.1038/s41580-021-00425-y
Share this article
Anyone you share the following link with will be able to read this content:
Sorry, a shareable link is not currently available for this article.
Provided by the Springer Nature SharedIt content-sharing initiative
This article is cited by
The spliceosome-associated protein cwc15 promotes mirna biogenesis in arabidopsis.
- Bangjun Zhou
Nature Communications (2024)
A long non-coding RNA functions as a competitive endogenous RNA to modulate TaNAC018 by acting as a decoy for tae-miR6206
- Qian-Huan Guo
Plant Molecular Biology (2024)
MicroRNA: A Novel Micro-machineries to Target Crop Plants for Tolerance to Temperature Stress
- Javaid Ashraf Nowshehri
- M. Ashraf Bhat
Plant Molecular Biology Reporter (2024)
Integrated analysis of transcriptome and small RNAome reveals regulatory network of rapid and long-term response to heat stress in Rhododendron moulmainense
- Li-Juan Xie
Planta (2024)
Microbe-induced gene silencing explores interspecies RNAi and opens up possibilities of crop protection
- Rongxiang Fang
Science China Life Sciences (2024)
Quick links
- Explore articles by subject
- Guide to authors
- Editorial policies
Sign up for the Nature Briefing newsletter — what matters in science, free to your inbox daily.

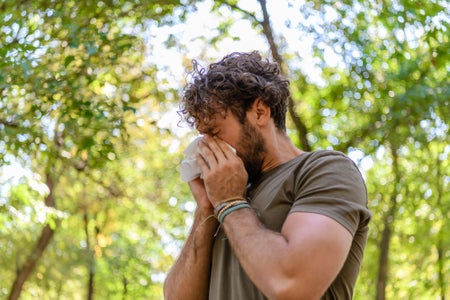
Why Seasonal Allergies Are So Miserable
Plants are just trying to reproduce; immune systems are just trying to keep us safe
Meghan Bartels
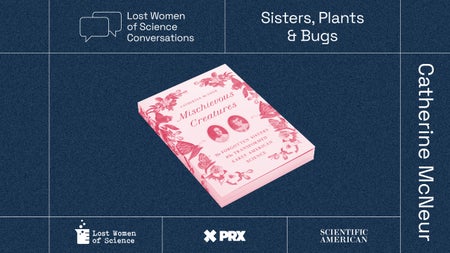
The Forgotten Sisters Who Transformed Early American Science
The Morris sisters made significant contributions to botany and entomology, but their stories were erased from the history of early American science, both accidentally and by design.
Katie Hafner, Catherine McNeur, Michelle Nijhuis, The Lost Women of Science Initiative
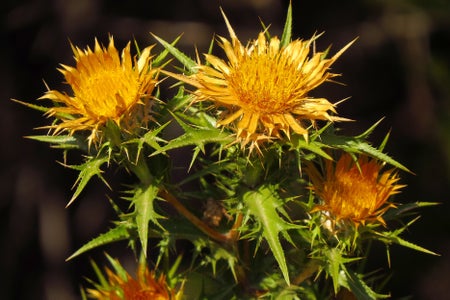
This Flower Refrigerates Itself to Survive Scorching Summers
A humble thistle blossom in southern Spain somehow keeps itself up to 18 degrees Fahrenheit cooler than the surrounding air
Elizabeth Anne Brown
This Genetically Engineered Petunia Glows in the Dark and Could Be Yours for $29
The engineered “firefly petunia” emits a continuous green glow thanks to genes from a light-up mushroom
Katherine Bourzac, Nature magazine
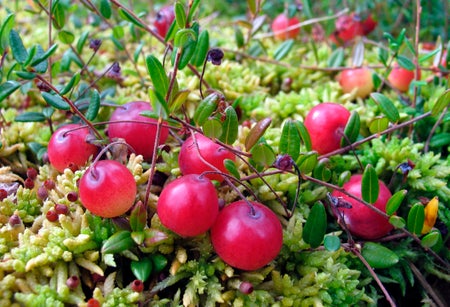
Cranberries Are a Scientific Delicacy
From self-pollination to bogs, cranberries are a Thanksgiving classic with many fascinating botanical and genetic features
Serina DeSalvio, The Conversation US
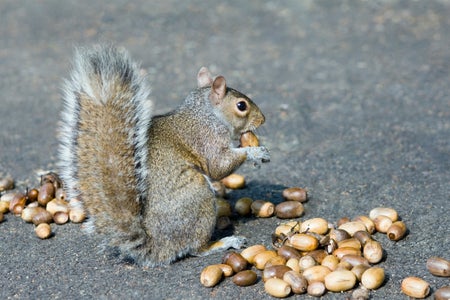
This Fall Is Full of Acorns—Thanks to a ‘Mast’ Year
Trees can outsmart animals such as squirrels and birds by synchronizing their seed production
Emily Moran, The Conversation US
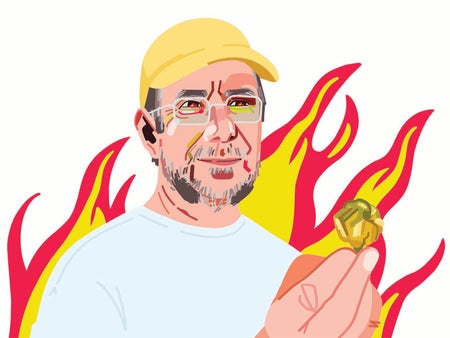
How Hot Is ‘Pepper X’? Its Creator Spent 6 Hours Recovering from Eating It
“Pepper X” is officially the hottest pepper in the world, weighing in with 2.693 million Scoville heat units. The creator reveals his process and experience tasting the pepper
Stephanie Pappas
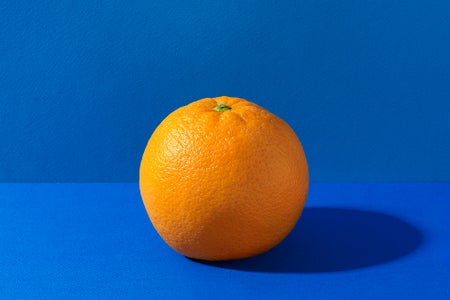
We Finally Know Where Oranges and Lemons Come From
In addition to finding where citrus come from, researchers have pinpointed the genetic origins of the fruits’ tart taste
Jack Tamisiea
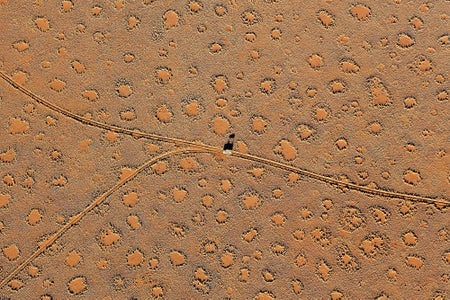
Thousands More Puzzling ‘Fairy Circles’ Have Been Found around the World
These mysterious spots of barren soil have fascinated scientists for years. Now evidence of their existence beyond two known locations is stirring up a fresh round of contention
Lori Youmshajekian
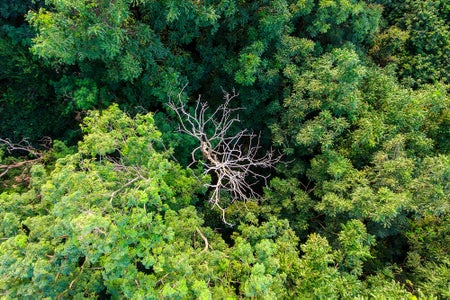
Tropical Forests May Be Getting Too Hot for Photosynthesis
When trees get too hot, energy production in their leaves breaks down
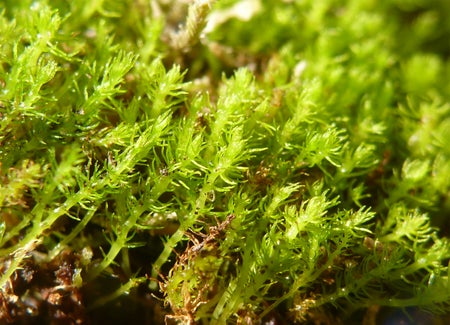
The World’s Oldest Moss Outlived the Dinosaurs, but It May Not Survive Climate Change
The world’s oldest moss has survived Earth’s shifting landscapes for more than 400 million years, but climate change is happening faster than it can adapt
Chelsea Harvey, E&E News
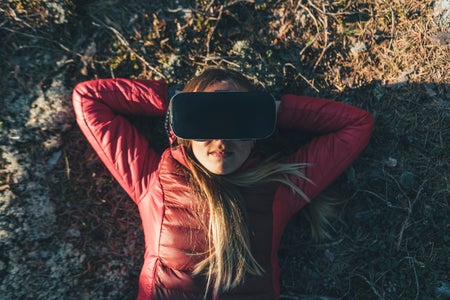
Can Virtual Reality Mimic Nature’s Power to Make Us Healthier?
Just seeing nature in VR brings some health benefits—and helps scientists learn why we need the real thing

An official website of the United States government
The .gov means it’s official. Federal government websites often end in .gov or .mil. Before sharing sensitive information, make sure you’re on a federal government site.
The site is secure. The https:// ensures that you are connecting to the official website and that any information you provide is encrypted and transmitted securely.
- Publications
- Account settings
Preview improvements coming to the PMC website in October 2024. Learn More or Try it out now .
- Advanced Search
- Journal List
- CBE Life Sci Educ
- v.13(3); Fall 2014
Seeing the Forest and the Trees: Research on Plant Science Teaching and Learning
Diane ebert-may.
*Department of Plant Biology, Michigan State University, East Lansing, MI 48824
† Department of Biology, Utah Valley University, Orem, UT 84058
In this editorial we link the articles published in this Special Focus section with the practical utility of using plants in education to transform and transcend traditional botany classes. We suggest current and future implications of research in this area.
Welcome to this special focus section of CBE—Life Sciences Education . A large portion of this issue is dedicated to investigations that explore how students think about plants and how faculty teach about plants. To date, people with an interest in studying plant science teaching and learning have limited journals for publishing their scholarly work. The call for contributions to this section was intended to attract authors and readers with an interest in plant science education. During the peer review process, one criterion for selection of papers required that the work provided insight into student learning focused specifically on plants. So, if plants were used as a possible example rather than as a focal point of learning, the paper was not considered appropriate for this section. Some very good instruction makes use of plants as examples, but we wanted to highlight learning challenges and opportunities unique to teaching and learning about plants.
Plants have long inspired influential individuals (e.g., Robert Hooke, Carl Linnaeus, Charles Darwin, Gregor Mendel, Barbara McClintock) whose contributions to science extend far beyond their botanical training. Today, one tiny mustard, Arabidopsis thaliana , has taken the world by storm. Uniting researchers across the globe, Arabidopsis transcends a model and has become the reference organism addressing major questions in plant biology in less than a quarter century. It has ushered in an explosion of molecular data as the first plant to have its entire genome sequenced; and it has served as the cornerstone for biotechnological applications with plants. Furthermore, Arabidopsis has been used in classrooms to improve learning of genetics ( Zheng, 2007 ), evolution ( Wyatt and Ballard, 2007 ), and biotechnology ( Barnard et al. , 2006 ), and to promote student research experiences ( Dolan et al. , 2008 ; Burnette and Wessler, 2013 ).
Beyond this single species, plants represent a system that does much more than photosynthesis, which is often the only plant concept taught in introductory courses. Research on plant systems is expanding, with implications for human health and ecosystem services—from climate change and bioenergy to drug discovery and food science. Plants do many interesting things at multiple scales that include, to mention a few, complex secondary metabolism, gene regulation, genomic evolution (while animals simply move in response to their environment), and carbon fixation for biomass to feed the world. We must move plant science education into the 21st century. Thinking of plants at multiple scales requires understanding of core ideas (evolution and ecology), cross-disciplinary concepts (matter and energy), and use of science practices (models, arguments, and cooperative work).
Using plant systems can help transform traditional descriptive botany courses into fully integrated plant science, while not losing the “trees in the forest.” For example, we posit that learning the names of plants is not simply memorization; rather, the knowledge and skills gained through this process can promote inquiry into community structure and function and foster an intimacy between a student and nature. On the practical side, compared with animals, plants are often less expensive, more scalable, and more flexible in the classroom, owing to the short generation time of some species and ease of avoiding ethical and safety constraints. Despite the accessibility of plants in promoting education in the sciences, research on curriculum and cognition clearly illustrates considerable bias by students and faculty in favor of their mobile, heterotrophic counterparts.
This special section attempts to highlight several types of research in plant sciences education. Three of these six articles report on original inquiry-based instructional materials to teach about what determines variation in phenotype, focusing on the inheritance and expression of quantitative traits using plants (Batzli et al .); how students connect science practices, explanations, and arguments from evidence about plant metabolism and growth (Dauer et al .); and the use of a computer-based program to learn plant identification (Kirchoff et al .). Two articles explore the conceptual foundations of “plant blindness”—the idea that students fail to notice or appreciate plants—among young children (Anderson et al .) and university students (Balas and Momsen). The final article reports how the infusion of plant-based research into majors’ courses affects students’ conceptual understanding, abilities to conduct and communicate research, and interest in plant science (Ward et al .).
We hope this body of work is both enjoyable and inspirational, promoting ideas for transformation of courses and stimulating future research. Often, animal or bacterial systems are taught as the exemplar, and students are expected to transfer that knowledge to plant systems. We assert that learning concepts about plant systems first may benefit students in two ways. First, due to their aforementioned unique properties (e.g., autotrophy), we propose that the knowledge and practices students gain from studying plant systems may be more portable into simpler heterotrophic systems, rather than vice versa. Second, using plants as the standard to teach basic biological principles may also combat documented “plant blindness.” This leads to the questions: What about plant science learning is transferable? How does transformed course design influence students’ understanding of plant concepts and science practices? The field is wide open for rich descriptions about implementation of plant science curricula and, importantly, evaluation of educational innovations. Does course transformation focused on plant science make a difference? What can students do better now than they did before? Finally, plant blindness exists—we need to know the cognitive underpinnings and instructional design that enables students to “see” plants and their applications and implications.
- Barnard B, Sussman M, Splinter BonDurant S, Nienhuis J, Krysan P. Microarrays (DNA chips) for the classroom laboratory. Biochem Mol Biol Educ. 2006; 34 :355–359. [ PubMed ] [ Google Scholar ]
- Burnette JM, Wessler SR. Transposing from the laboratory to the classroom to generate authentic research experiences for undergraduates. Genetics. 2013; 193 :367–375. [ PMC free article ] [ PubMed ] [ Google Scholar ]
- Dolan EL, Lally DJ, Brooks E, Tax FE. PREPping students for authentic science. Sci Teach. 2008; 75 :38–43. [ PMC free article ] [ PubMed ] [ Google Scholar ]
- Wyatt S, Ballard HE. Arabidopsis ecotypes: a model for course projects in organismal plant biology and evolution. Am Biol Teach. 2007; 69 :477–481. [ Google Scholar ]
- Zheng Z-L. Use of the gl1 mutant and the CA-rop2 transgenic plants of Arabidopsis thaliana in the biology laboratory course. Am Biol Teach. 2007; 68 :e148–e153. [ PMC free article ] [ PubMed ] [ Google Scholar ]
Plant and Animal Reproduction
While all organisms reproduce, not all organisms reproduce the same way. Explore the similar and different ways that plants and animals pass on their genes.
Biology, Genetics
Loading ...
All organisms reproduce, which is the biological process where an organism produces and/or gives birth to another organism. Both plants and animals reproduce, though they have evolved the processes so that they overlap and diverge from each other in several ways. Types of Reproduction There are two types of reproduction: asexual reproduction and sexual reproduction . A sexual reproduction involves a single parent that produces a genetically identical offspring. Sexual reproduction involves two parents of the opposite sex. A male plant or animal contributes genetic material in the form of sperm or pollen to a female plant or animal's egg. The offspring then has genetic material from both parents. Different plants and animals can reproduce either asexually or sexually; however, a sexual reproduction is more common among plants than animals. Asexual and sexual reproduction each have benefits and drawbacks. Organisms that reproduce asexually have the advantage of producing several genetically identical offspring quickly and with little energy. On the other hand, the lack of genetic diversity among asexual offspring means they have a lower chance of adapting to an unstable environment. By contrast, organisms that reproduce sexually have the advantage of producing a genetically diverse offspring, which is able to adapt to its environment. But sexual reproduction comes at a cost, requiring more time and energy to produce an offspring than a sexual reproduction . Asexual Reproduction There are a variety of ways plants can reproduce asexually, or without a partner. For example, some nonflowering plants, such as moss and algae, reproduce by spore formation. Spores grow on a plant, then break off and grow into separate organisms. Other plants, such as strawberries, are able to reproduce asexually through vegetative propagation . This process involves using a part of a plant, such as a root or stem, to produce a new plant, and can happen either naturally or artificially. Other artificial methods, such as grafting , involve combining two plants into one by attaching the top part of a plant, called a scion , to the lower part of a plant, called a rootstock . Sexual Reproduction and Fertilization Many plants and most animals require partners to reproduce. Plants and animals share their genetic material in a process called fertilization . In plants, fertilization happens when the male shares pollen , which contains its genetic material, with a female plant's egg. In flowering plants, an egg is fertilized by cross- pollination . This process often requires an insect, such as a bee, that transfers grains of pollen from the male part of a flower, which is called the anther , to the female part of a flower, which is called the stigma . Once the pollen lands on the stigma , it passes through a long, tube-like structure called a style to reach the plant's ovaries. This part of the reproductive organ is where fertilization takes place. Some plants, called hermaphrodites , have male and female parts on the same plant, and are able to self-pollinate. Animals, by contrast, do not depend on third parties like insects for fertilization . As mobile creatures, animals can directly transfer sperm to an egg by physically interacting with each other. They often perform various mating rituals in order to attract a potential partner. Embryonic Development Once a plant or animal egg is fertilized, it starts developing into a multicellular organism. During this early stage, the fertilized egg is called an embryo. Despite differences in the fertilization process, the development of plant and animal embryos is similar. A plant embryo is contained within a seed, which provides the nutrients it needs to grow, while an animal embryo develops within an egg, outside the organism, or within a uterus, inside the female parent organism. Birth and Germination Plants and animals also differ with respect to how they give birth. Animals exit the female's uterus as a newborn or hatch from an egg that has already left the female's body. A plant, by contrast, begins its life by sprouting from a seed. The plant releases the seed, which begins to grow once it is in the soil and the conditions are right. After the seed has sprouted into a plant, it can collect additional nutrients through its roots.
Media Credits
The audio, illustrations, photos, and videos are credited beneath the media asset, except for promotional images, which generally link to another page that contains the media credit. The Rights Holder for media is the person or group credited.
Production Managers
Program specialists, last updated.
October 19, 2023
User Permissions
For information on user permissions, please read our Terms of Service. If you have questions about how to cite anything on our website in your project or classroom presentation, please contact your teacher. They will best know the preferred format. When you reach out to them, you will need the page title, URL, and the date you accessed the resource.
If a media asset is downloadable, a download button appears in the corner of the media viewer. If no button appears, you cannot download or save the media.
Text on this page is printable and can be used according to our Terms of Service .
Interactives
Any interactives on this page can only be played while you are visiting our website. You cannot download interactives.
Sciencing_Icons_Science SCIENCE
Sciencing_icons_biology biology, sciencing_icons_cells cells, sciencing_icons_molecular molecular, sciencing_icons_microorganisms microorganisms, sciencing_icons_genetics genetics, sciencing_icons_human body human body, sciencing_icons_ecology ecology, sciencing_icons_chemistry chemistry, sciencing_icons_atomic & molecular structure atomic & molecular structure, sciencing_icons_bonds bonds, sciencing_icons_reactions reactions, sciencing_icons_stoichiometry stoichiometry, sciencing_icons_solutions solutions, sciencing_icons_acids & bases acids & bases, sciencing_icons_thermodynamics thermodynamics, sciencing_icons_organic chemistry organic chemistry, sciencing_icons_physics physics, sciencing_icons_fundamentals-physics fundamentals, sciencing_icons_electronics electronics, sciencing_icons_waves waves, sciencing_icons_energy energy, sciencing_icons_fluid fluid, sciencing_icons_astronomy astronomy, sciencing_icons_geology geology, sciencing_icons_fundamentals-geology fundamentals, sciencing_icons_minerals & rocks minerals & rocks, sciencing_icons_earth scructure earth structure, sciencing_icons_fossils fossils, sciencing_icons_natural disasters natural disasters, sciencing_icons_nature nature, sciencing_icons_ecosystems ecosystems, sciencing_icons_environment environment, sciencing_icons_insects insects, sciencing_icons_plants & mushrooms plants & mushrooms, sciencing_icons_animals animals, sciencing_icons_math math, sciencing_icons_arithmetic arithmetic, sciencing_icons_addition & subtraction addition & subtraction, sciencing_icons_multiplication & division multiplication & division, sciencing_icons_decimals decimals, sciencing_icons_fractions fractions, sciencing_icons_conversions conversions, sciencing_icons_algebra algebra, sciencing_icons_working with units working with units, sciencing_icons_equations & expressions equations & expressions, sciencing_icons_ratios & proportions ratios & proportions, sciencing_icons_inequalities inequalities, sciencing_icons_exponents & logarithms exponents & logarithms, sciencing_icons_factorization factorization, sciencing_icons_functions functions, sciencing_icons_linear equations linear equations, sciencing_icons_graphs graphs, sciencing_icons_quadratics quadratics, sciencing_icons_polynomials polynomials, sciencing_icons_geometry geometry, sciencing_icons_fundamentals-geometry fundamentals, sciencing_icons_cartesian cartesian, sciencing_icons_circles circles, sciencing_icons_solids solids, sciencing_icons_trigonometry trigonometry, sciencing_icons_probability-statistics probability & statistics, sciencing_icons_mean-median-mode mean/median/mode, sciencing_icons_independent-dependent variables independent/dependent variables, sciencing_icons_deviation deviation, sciencing_icons_correlation correlation, sciencing_icons_sampling sampling, sciencing_icons_distributions distributions, sciencing_icons_probability probability, sciencing_icons_calculus calculus, sciencing_icons_differentiation-integration differentiation/integration, sciencing_icons_application application, sciencing_icons_projects projects, sciencing_icons_news news.
- Share Tweet Email Print
- Home ⋅
- Science ⋅
- Nature ⋅
- Plants & Mushrooms
Characteristics of Plants & Animals
What Are the Three Main Differences Between a Plant Cell and an ...
Plants and animals are both living things, but at first glance, they seem very different. Animals tend to move around, while plants stay rooted in one place. Animals eat their food, while plants convert sunlight into the energy they need. Despite these differences, scientists argue that plants and animals are more similar than they are different. Some living things even blur the line between the plant and animal kingdoms.
TL;DR (Too Long; Didn't Read)
Plants and animals share many characteristics, but they are different in some respects. Animals usually move around and find their own food, while plants are usually immobile and create their food via photosynthesis. Plants and animals both have cells that contain DNA, yet the structure of their cells differs. Animal cells absorb nutrients from food, while plant cells use plastids to create energy from sunlight.
Plant and Animal Cellular Structure
Because both plants and animals are living things, they have cells. Cells are the smallest functional units of living organisms, and they make up every part of organism bodies. In some ways, plant and animal cells are similar. In others, they are vastly different.
Both plant and animal cells carry DNA – genetic material that is passed down from one generation to another. Because of DNA, plants and animals can pass on their genes over time and adapt to the environment around them via natural selection. Plant and animal cells both divide. Cell division is how individual animals and plants grow and replace parts of themselves. Human children reach adult height because of cell division, and grass grows for the same reason. Both plant and animal cells absorb nutrients and convert those nutrients into usable energy. Animal cells absorb nutrients from food, while plant cells absorb energy from sunlight via a process called photosynthesis.
Plant and animal cells have their differences, however. Plant cells are surrounded by a stiff cell wall, which helps keep plants rigid and upright, while animal cells are surrounded by a thin, permeable membrane that permits the absorption of outside substances. Plant and animal cells also contain differing organelles – inner-cellular structures. Some animal cells have cilia, the hairlike protrusions that help the cell move around. Plant cells do not have cilia, although most plant cells contain plastids. These organelles, which animal cells lack, contain pigment or food and are necessary for photosynthesis.
Plant and Animal Senses
Human beings have five senses: sight, scent, taste, touch and hearing. In fact, all living things, including plants, have senses, but without eyes, noses, tongues, skin or ears, can plants even sense the world around them? The answer is yes. All living things can sense the world around them, although they do so in different ways.
Most animals have fairly complex central nervous systems. Vertebrates – animals with a brain and spinal cord, such as human beings – have especially developed senses. Even invertebrates usually possess all or most of the five basic senses. Animals' bodies interpret light, chemical signals, pressure and sound waves to understand what is going on around it.
Plants sense their environment in other ways. Instead of sensory organs, they use a combination of hormones and sensory ions to take in information. Plants can sense light, which is important since sunlight is a plant's main source of energy. Plants slowly move over time to lean toward sunlight. Plants can also sense when the sun goes down. Scientists have found that certain plant species open pores on their leaves during the day to take in maximum sunlight, but close the pores at night to prevent moisture loss. Scientists have recently discovered that plants can even communicate with one another. About 90 percent of plants have mutually beneficial relationships with fungus, which spreads out underground in large webs. These webs can link the roots of several plants together, allowing the plants to send signals and nutrients back and forth. Plants may send beneficial carbon to their neighbors via the "fungal" network or even toxic chemicals if new, competing plants begin to sprout.
Plant or Animal?
Usually, it is easy to tell a plant from an animal simply by looking. Animals move around and find their food. Plants are immobile and create their food. However, some creatures blur the line between plant and animal. These creatures possess characteristics that make classifying them as plants or animals difficult.
For example, coral reefs are colorful, underwater gardens located in warm ocean waters. The coral itself appears rooted in place, entirely immobile. In shades of green, pink and yellow, with round or petal-like shapes, coral resembles flowers. In almost every way, coral looks and behaves like a plant. However, coral is an animal that gathers its own food. Coral reefs are created by millions of tiny coral polyps clustered together, excreting an exoskeleton base to which they cling.
Venus flytraps, easily identified as plants by their green leafy appearance, exhibit behavior that is usually reserved for animals. These plants have "mouths" that clamp shut when insects land inside. The Venus flytrap even lines its mouth pad with a sweet-smelling substance to draw flies and other bugs. Whether this counts as hunting is up for debate, but there is no doubt that Venus flytraps move and eat food in addition to creating energy from sunlight via photosynthesis. Almost no other plants do this.
With thick "stems," bright colors and waving "petals," sea anemones look like beautiful ocean flowers swaying with the tide. At first glance, they appear to be plants, but these creatures are animals, and over periods of days or weeks, they can travel short distances.
Plants and animals have many differences, but many similarities as well. Some animals are so similar to plants and vice versa that they can be difficult to classify at first sight. All living creatures, plants and animals alike, share a common ancestor, which means that we are all related, in spite of the differences in our cells and senses.
Related Articles
What are the three main differences between a plant..., how are fungi & plants similar, comparison of plant cells & human cells, do plant cells have flagella, levels of cell organization, adaptations of ocean plants, how to compare the cells of plants, animals & unicellular..., why plant intelligence is real, interesting facts about plant cells, why is breathing important to organisms, six basic parts of a plant, importance of aerobic cellular respiration, water lily adaptations, how does a plant convert light energy to chemical energy, preschool lessons that teach the parts of a flower, how do plants make their own food, how to make a 3d model plant cell without food, what are the characteristics of the protista kingdom, what is glucose made of, how to compare a frog and a human respiratory system.
- Fairfax County Public Schools: Classification of Organisms
About the Author
Maria Cook is a freelance and fiction writer from Indianapolis, Indiana. She holds an MFA in Creative Writing from Butler University in Indianapolis. She has written about science as it relates to eco-friendly practices, conservation and the environment for Green Matters.
Find Your Next Great Science Fair Project! GO
- Tools and Resources
- Customer Services
- African Literatures
- Asian Literatures
- British and Irish Literatures
- Latin American and Caribbean Literatures
- North American Literatures
- Oceanic Literatures
- Slavic and Eastern European Literatures
- West Asian Literatures, including Middle East
- Western European Literatures
- Ancient Literatures (before 500)
- Middle Ages and Renaissance (500-1600)
- Enlightenment and Early Modern (1600-1800)
- 19th Century (1800-1900)
- 20th and 21st Century (1900-present)
- Children’s Literature
- Cultural Studies
- Film, TV, and Media
- Literary Theory
- Non-Fiction and Life Writing
- Print Culture and Digital Humanities
- Theater and Drama
- Share This Facebook LinkedIn Twitter
Article contents
Plants and literature.
- Susan McHugh Susan McHugh University of New England
- https://doi.org/10.1093/acrefore/9780190201098.013.1267
- Published online: 31 August 2021
In countless ways, plants have been in literature from the start. They literally provide surfaces and tools of inscription, as well as figuratively inspire a diverse body of writing that ranges from documenting changing social and ecological conditions to probing the limits of the human imagination. The dependence of human along with all other life on vegetal bodies assures their omnipresence in literatures across all periods and cultures, positioning them as ready reference points for metaphors, similes, and other creative devices. As comestibles, landscape features, home décor, and of course paper, plants appear in the pages of virtually every literary text. But depictions of botanical life in action often prove portentous, particularly when they remind readers that plants move in mysterious ways. At the frontiers of ancient and medieval European settlements, the plant communities of forests served as vital sources of material and imaginative sustenance. Consequently, early modern literature registers widespread deforestation of these alluring and dangerous borderlands as threats to economic and social along with ecological flourishing, a pattern repeated through the literatures of settler colonialism. Although appearing in the earliest of literatures, appreciation for the ways in which plants inscribe stories of their own lives remains a minor theme, although with accelerating climate change an increasingly urgent one. Myths and legends of hybrid plant-men, trees of life, and man-eating plants are among the many sources informing key challenges to representing plants in modern and contemporary literature, most obviously in popular genre fictions like mystery, horror, and science fiction (sf). Further enlightening these developments are studies that reveal how botanical writing emerges as a site of struggle from the early modern period, deeply entrenched in attempts to systematize and regulate species in tandem with other differences. The scientific triumph of the Linnaean “sexual system” bears a mixed legacy in feminist plant writing, complicated further by Black, Indigenous, and People of Color (BIPOC) writers’ creative engagements with the unevenly felt consequences of professionalized plant science. Empowered by critical plant studies, an interdisciplinary formation that rises to the ethical challenges of emergent scientific affirmations of vegetal sentience, literature and literary criticism are reexamining these histories and modeling alternatives. In the early 21st century with less than a fraction of 1 percent of the remaining old growth under conservation protection worldwide, plants appear as never before in fragile and contested communal terrains, overshadowed by people and other animals, all of whose existence depends on ongoing botanical adaptation.
- botanical criticism
- critical plant studies
- ecocriticism
- literary and cultural plant studies
- phytocriticism
- phytocentric criticism
- phytographia
- phytopoetics
- plant thinking
- vegetal poetics
Plants and literature are both generic terms for highly varied and evolving referents. While plants permeate literary texts across all periods and cultures, their significance to literary critics remains uneven. In the works of the Bard alone, literary glossaries and lexicons from Henry Ellacombe’s Plant-Lore and Garden-Craft of Shakespeare ( 1878 ) through Gerit Quealy and Sumie Haegawa Collins’s Botanical Shakespeare ( 2017 ) register longstanding interest in the mentions, motifs, and of course plant-based potions used as plot devices. Apart from utilitarian objects, however, representing the lives of plants—ethically, culturally, politically, historically, philosophically, and textually—has proven more challenging to literary writers, a problem that fuels a fast-growing subfield of scholarship in the 21st century .
All accounts of why plants are moving from the margins to the center of literary study point to the cross-pollinations made possible through interdisciplinary studies. William Shakespeare’s poisons notwithstanding, the rise of medical humanities has renewed interest in rhetorical and philological approaches to plants, particularly in classical and medieval scholarship, where substantial bodies of herbal-healing literature survive. In literary animal studies, the very different claim that plants are the final frontier of post-anthropocentric critique stirs controversy. Still more ambivalence surrounds the languishing of botanical interests following Lawrence Buell’s turn-of-the- 21st-century heralding of a definitive “environmental turn in literary-critical studies,” sparking debates about how literary writing creates, disseminates, and subverts ecocritical perspectives on plants as agentive beings. 1 Wider-ranging studies of the intertwining histories of literature and plant science are revealing the radical potentials that stem from the intersections of botanical knowledges and forms of expressing them, along with their proliferations and contestations through histories of settler colonialism. Elaborating the uneven consequences for people and plants, recent feminist, antiracist, and decolonial writing illustrates unique possibilities for literature in critical plant studies, a field gaining legitimacy through questioning how plants came to be seen as inert, insensate, and unintelligent.
Critical plant studies aims to inspire more comprehensive and sympathetic accounts of the implications of growing new evidence of the complex intra- and inter-species subterranean, biochemical, and airborne communications shared among photosynthesizing and saprophagous life forms. Philosopher Michael Marder elaborates how “plant thinking” is never simply a question of vegetal capacities: what humans think about plants can be distorted by metaphysical biases that compound our difficulties with perceiving vegetal sentience. Marder draws together insights from phenomenology, botany, and population ecology to model ways of ruminating that “neither treat . . . plants as passive objects (or quasi-mechanical structures relegated to the background of animal life) nor accept . . . the Western metaphysical equation of subjectivity with autonomy, unity, individuality, personhood or will.” 2 His prescient framing of critical plant studies as “an interdisciplinary dialogue, whereby philosophy and literature would learn from each other to think about, imagine, and describe vegetal life with critical awareness, conceptual rigor, and ethical sensitivity” drives inquiry into how literary workspaces have always been sites of struggle to articulate what is distinctive to botanical being-in-the-world. 3 Thus plant life as such no longer marks a literary-historical limit but rather becomes an inspiration for representing and recovering ethically driven plant-human relations in literature.
Getting deeper into the weeds of literary history highlights the profoundly different genealogies of critical plant studies and ecosophy. Following Marder’s lead by tracking leading-edge science, Molly Mahood’s The Poet as Botanist ( 2008 ) traces how poets’ seemingly endless fascination with flowers changed with the publication of Swedish botanist Carolus Linnaeus (Carl von Linné)’s Systema Naturae ( 1735 ), which simultaneously revolutionized the study of botany and language. Through “the close relationship of poetry and plant science” that “held ever since Linnaeus,” Mahood charts how organismal botany rose to fame. But, by the mid- 20th century , the popularity of botany became imperiled by increasingly technical interests through the rise of “biochemistry and molecular biology, . . . return[ing only] through the back door as plant ecology.” 4 A comparative view of literary history casts even more doubt on the inevitable or inherent ecocritical appeal of plants.
The systematic collection and study of plants empowered by European colonialism led not just to more imaginative literary writing through science but also established mutual influences. Best known for literary works like The Sorrows of Young Werther ( 1774 ), Johann Wolfgang von Goethe authored two publications that share the title Metamorphose der Pflanzen ( Metamorphosis of Plants )—the first a botanical treatise ( 1790 ) and the next an elegiac poem ( 1798 )—that together reflect how literary and scientific imaginations were mutually transformed by the Linnaean emphasis on shared morphological traits. Discovering how every tree is encapsulated by a meristem of embryonic tissue, which inscribes every experience of their lives in their own wood, is tantamount to recognizing how morphology makes of plants “a metaphor of metaphors”: open-ended, receptive, wondrous. 5 Goethe contemporary and friend Alexander von Humboldt’s even more widely influential Essai sur le Géographie des Plantes ( 1804 ) is credited as a foundational text of ecology and biogeography, and also as an aesthetically innovative textual interweaving of images and words. 6 Such works also mark profound transitions away from plants as objects of the classifying imagination and toward vegetal beings’ capacities for endless growth, inciting revolutionary thinking about questions of representation, broadly writ.
In the same period, Erasmus Darwin’s The Botanic Garden ( 1791 ) more directly synthesizes scientific and literary writing by binding together two poems in a single volume: “The Economy of Vegetation,” geared to introduce a general readership to a range of scientific questions of the day; and “The Loves of the Plants” (initially published in 1789 ), which capitalized on Linnaeus’s sexually explicit language to advance evolutionary thinking about human-plant continuities. Yet the complex potentials and limits of women’s affiliations with plants also contributed to the book’s sensational response, as Sam George elaborates in Botany, Sexuality, and Women’s Writing, 1760–1830: From Modest Shoot to Forward Plant ( 2007 ), which tracks the influence of the Linnaean sexual system on science and literature by focusing on the pivotal role of Darwin’s book in British women’s education. Through scenes pandering to misogynist (some also racist) fantasies, “The Loves of the Plants” pointedly challenged established assumptions that botany and floriculture were respectable foci for a lady’s education; girls’ and women’s rare point of access to scientific training consequently came under threat. The influence of Darwin’s poem on literary production was no less profound, particularly for women writers, who were both inspired by and scrutinized for engaging with the vitality of female plant parts. 7 Art imitating life, Elizabeth Gilbert’s The Signature of All Things ( 2013 ) provides a fictional meditation on how 18th - and 19th-century Euro-American women studying plants such as Jane Colden, Jeanne Baret, and Marianne North overcame obstacles to make science history as botanical researchers and explorers.
New materialist approaches to literary criticism are also helping to make literature’s material origins in plant life more apparent today. Through a reading of Abraham Cowley’s poem “Written in Juice of Lemmon” ( 1656 ), Julian Yates’s Of Sheep, Oranges, and Yeast: A Multispecies Impression ( 2017 ) swerves the materiality of media toward self-reflexivity, here in the form of secret writing in citrus juice on paper revealed through delicate application of flame. Cowley’s perspective highlights how “plants funded and framed acts of inscription,” according to Yates: “literally (and figuratively) growing, living out their own forms of being in and by and through their use as media that bound human expression,” the medium that is the message “fruiting as it appears” reveals the fruit as symbiotically inhabiting literary expression. 8 But can literary expressions also trace the perspectives of literal plants?
To move beyond deadlocked debates about whether or not plants can embody subjectivity or assert agency, critics are reorienting discussions of plant speech toward more precise literary preoccupations with plant language and writing. Patrícia Vieira advocates for building more directly on the extensive emerging knowledges of biochemical signaling and other forms of plant communication by centering literary interpretation on “phytographia,” or “imprints left in texts themselves by plants.” 9 Starting with 17th-century German mystic Jakob Böhme’s theory that “God’s signatures” are apparent in all things, Vieira dismantles hierarchic views of nonhuman languages still more decisively via Jacques Derrida’s concept of “arche-writing,” or a type of writing that precedes the subjugation of writing as a vehicle of speech and meaning. As a mode of plant inscription, phytographia enables deconstructions of the contradictions peculiar to plants and literature, and more. 10 Vieira’s studies of Amazonian plants’ inscriptions on human lives in Colombian and more recently Brazilian and Portuguese modernist “novels of the jungle” further suggest how attempts to represent the vegetal voices of the rainforest give rise to literary creations that resist superimpositions of human stories, along with their silencing through settler colonialism. 11
Cross-cultural comparisons of historical representations of plants are destabilizing distinctions between naturalist and literary writing. 12 Such discussions provide just a sample of how literature and literary studies alike are themselves profoundly changed by fostering what Joela Jacobs conceptualizes as “phytopoetics,” an aesthetics of plant life that upends especially passive stereotyping by attending to the ways in which plants’ life-giving and life-taking capacities are not transcended by so much as reasserted in literary writing, particularly about what she terms “vegetal eroticism” and “vegetal violence.” 13 The phytopoetic charge of the nascent subfield of literary and cultural plant studies is leading to recoveries and reconsiderations of how writers have taken up the creative challenges of representing plants on their own terms, often through distinct themes and genres.
The materiality of plants in the instruments, surfaces, and everyday lives of literary authors is readily apparent from ancient traditions worldwide, and anthropomorphized vegetal characters are among the oldest staples of folklore. By the 20th century , their refusal to keep still in the objectifying gaze makes botanical beings ripe for retooling as threats, particularly as mass menaces or plant horrors. In contrast, rare individual plants, particularly trees, trend more sympathetic, especially when cast as bearing witnesses to atrocities, in feminist, antiracist, queer, and postcolonial literatures. Mixed communities of plants anchor more ambivalent literary histories, flagged by forests gradually changing from sites of satire or refuge to spooky, if not downright dangerous, zones for vulnerable people. Plants play more direct roles in representations of agricultural communities in flux, prompting bucolic reflections in critiques of urbanization, and devastating visions of manufactured food shortages and other crises that follow from industrialization and consolidation of farming.
As sites of hybridization, chemical manipulation, and genetic modification of plants, laboratories link together many of these scenes and more: bridging the commercialization of plant life and the growing prominence of plant horror, creative representations of technical control also extend connections forged between literature and science in the European Enlightenment that again include botanical capacities for resistance. 14 The divergent perspectives of botanical scientists from others with vested interests in plant life—whether horticulturalists, plant developers, or hobbyists—are signaled by literary representations of plant life changing through natural and especially artificial selection in sites closer to home. Whether in gardens, greenhouses, or simply pots, vegetal silence begins to emerge as a sign of exclusions by as well as within human communities. Through the rising popularity of botanically oriented memoirs at the turn of the 21st century , writers as different as novelist Jamaica Kincaid and botanist Robin Wall Kimmerer are troubling histories of colonialism, enslavement, genocide, and extinction by finding ways of reconnecting with their heritage cultures, lands, languages, and losses through plants. These budding interests in ethically writing plant life indicate how engagements with botanical theory and horticultural practice continue to enable explorations of new frontiers in literary studies.
Plant Matter
The material presences of plants in writing make possible a play of sign and referent from literature’s inception. Carved with a blunt reed, the remaining fragments of the oldest literary text, The Epic of Gilgamesh ( c . 2100 bce ), include a story of the hero’s discovery and loss of a plant that would bring immortality. From ancient times, the plant matter of literary musing has included bark, bast, and other writing surfaces sourced from such diverse species as papyrus, flax, and fig trees. Of the hundreds of plants represented in the oldest Chinese literature, the mulberry is by far the most mentioned in Shijing or Classic of Poetry ( 1100–700 bce ). Writing long before the invention of paper and the lucrative trade in raw silk for textiles, its writers recognized mulberry trees as cultivated in plantations for the purpose of feeding the insect source of their primary writing surface: silk, usually used together with lacquer sourced in tree sap, was preferred before the invention of paper there. 15 Although virtually any plant can be used for papermaking, the exceptional durability of cotton-pulp paper makes it the standard for archiving literature, and along with it enduring material connections to the history of slavery. The detail in Toni Morrison’s novel Beloved ( 1987 ) that the slave Sethe is tasked with making the ink used by her tormentor Schoolteacher likewise serves as a subtle reminder of the forced labor behind another one of Euro-Americans’ main writing materials from the 14th through the 19th centuries : iron gall ink, sourced from oak trees. 16
Human dependence on plants in general and obligations to trees in particular are familiar themes within ancient literatures, for instance in the Sanskrit tradition extolling the virtues of the mystical Forest Queen or “mother of all sylvan things” in the Rigveda ( c . 1500 bce ) and outlining rules for cohabitating with plants in the Puranas ( c . 400–600 ). 17 To their sacred knowledges, symbolic meanings, and medicinal properties is added a mnemonic device in the early medieval Irish alphabet Ogham, to each letter of which is ascribed the name of a kind of tree. The world tree Yggdrassil of the Old Norse sagas exemplifies how individual plants even occasionally figure as powerful presences within the oldest texts.
Whether via metaphor, simile, or personification, the vegetal has long served literary authors as a ready ruse by which to say things that are unspeakable in human terms. In the gardener’s discussion scene in Shakespeare’s Richard II ( 1595 ), menial servants are overheard speaking of fruiting trees, herbs, ornamentals, and weeds in postlapsarian disarray, but immediately understood by the queen to be actually discussing the failures of the titular king to manage his subjects. Throughout the play, the overtaxing of lands and people alike are figured through botanical vocabularies that clarify the stakes of the king’s poor cultivation of all within his island nation, rather, “our sea-walled garden” (3.4.42), according to the gardener’s servant. 18 Settler nations’ dependence on coordinated cultivation of the land with the most nutritive plants for table and livestock may not immediately spring to playgoers’ minds, but the metaphor only works because the questions of stewardship raised in the castle garden are recognized as applying as much to statesmanship as they do to horticulture. 19
In contrast, botanical rhetorics in modern literatures trend toward rendering plant life unimportant, most obviously through dead vegetal metaphors. Bearing associations with Aphrodite and the Virgin Mary, the flower figured in Robert Burns’s famous opening line “O my Luve’s like a red rose” ( 1794 ) reflects the Romanticist enlistment of botanical subjects as vehicles for expressing strictly human emotions. Over a century later, Gertrude Stein’s trademark quip “A rose is a rose is a rose” will become a preeminent illustration of the modernist literary counter-valuation of literal language (the line itself echoing Stein’s 1913 poem “Sacred Emily”). However unthinkable literature may be without plant matter, vegetal life appears also to be a limit case for the properly literary subject, a condition amplified by the rising popularity of fictional vegetal monstrosities in modernity.
Plant Horror
Examples from contemporary realistic fiction around the world—including Ibrahim al-Koni’s The Bleeding of the Stone ( 2002 ), Richard Powers’s The Echo Maker ( 2006 ), and Han Kang’s The Vegetarian ( 2007 )—figure vegetal violence ironically, through the silent suffering of human characters who are victimized for being vegans or vegetarians. It’s a new twist on villainous vegetal representations in literary history, where botanical beings overwhelmingly assert themselves to the detriment of humans. And, when literary plants go bad, they do so often as mindless, monstrous menaces, in examples as diverse as the heroine’s poisonous vegetal “sisters” in Nathanael Hawthorne’s “Rappacini’s Daughter” ( 1844 ) and the mysteriously manipulative growth that haunts Jeff VanderMeer’s Annihilation ( 2014 )—examples that also use botanical figures to explore the demonization of female desire. While both leave open the question of plant culpability in the deaths of humans, there are far more literary plants that appear to crave human flesh, in “inscrutable silence and [with] an implacable strangeness.” 20
The roots of plant horror reach back to carnivorous variations on legends of the zoophyte known as the borametz , or vegetable lamb of Tartary, said in some variations to consume all who venture within its soil-rooted range. 21 Eighteenth-century European reports of the Javanese upas tree having a lethal poison that seeps through the ground and travels airborne (along the lines of the film The Happening , 2008 ) were only scientifically debunked by the mid- 19th century , by which time the dream of killer plants had become firmly rooted in the gothic imaginary. 22 Ranging wider away from colonial centers, the passive floral poisoner of Hawthorne’s story morphs into a more active threat. 23 As early as 1874 , a report began to circulate about an “atrocious cannibal tree” fed by tribal sacrifice in Madagascar, later outed as the cryptobotanical brainchild of fiction writer Edmund Spencer. 24 Published worldwide as a news story, its momentum is attributable to the coincidental publication of Charles Darwin’s Insectivorous Plants ( 1875 ), which confirms that some plants can have humanlike digestive mechanisms. Although Darwin clarifies that such plants are native to all continents except Antarctica, racialized fantasies of jungle-dwelling peoples’ proximities to carnivorous plants persist, for instance, in Scott Smith’s novel ( 2006 ) and film ( 2008 ) The Ruins , which portrays white Euro-American travelers falling victim to a parasitic vine at an overgrown temple tended by Maya people.
Written with his son Francis, Darwin’s later book The Power of Movement in Plants ( 1880 ) advanced further the hypothesis that plants demonstrate cognition, based on evidence that they act according to their appetites, a potential that becomes decidedly sinister in the literary imagination. Dramatic thrills and chills move with botanical specimens into the centers of empire through flesh-eating plants depicted as individuals collected and eventually bred by horticulturalist men, including the titular specimens of H. G. Wells’s “The Flowering of the Strange Orchid” ( 1894 ) and Arthur C. Clarke’s “The Reluctant Orchid” ( 1954 ). Such stories also satirize the 19th-century eruption of “orchidelirium,” a variant on the Dutch Golden Age “tulipmania,” in which plant-collecting passions tip over into self-destructive obsessions. 25 Charles Addams’s Cleopatra, a member of the fictitious African Strangler species and the prize of fictional Addams-family-matriarch Mortitia’s exotic collection of deadly plants, is a prominent indicator of how the pattern of racial and ethnic coding of botanical killers persists well into the 20th century . That said, named individuals like Audrey II of Little Shop of Horrors ( 1960 , 1986 ) fame, along with the carnivorous titular character of Annie Proulx’s “The Sagebrush Kid” ( 2008 ), however deadly, are likewise plants deliberately nourished by humans as symbionts, even family.
Audrey II’s alien origins and monstrous proportions also reflect the ways in which the trope of vegetal attacks on humans develops through Cold War discourses of invasion. In the 20th century , fictions-turned-films like The Body Snatchers ( 1955 ) / Invasion of the Body Snatchers ( 1956 ; 1978 ) hearken back to radical traditions in botanical writing that challenge human dominance even while gaining in popular currency. The spectacle of ambulatory alien plants intentionally poisoning people in John Wyndham’s The Day of the Triffids ( 1951 ) inspired a radio series ( 1960 ), a film ( 1962 ), a TV series ( 1981 ), even a sequel in the form of Simon Clark’s novel The Night of the Triffids ( 2001 )—a phenomenon that gains greater significance amid the struggle to advance nonanthropocentric ecological thinking. Even as debates grow about the literary values of botanical resistance—whether it figures fights for ecology, or against sexual, ethnic, or racial inequality—the tendency to interpret plants as stand-ins for humans in the history of literary criticism helps to explain why the need to read them on their own terms becomes felt so strongly.
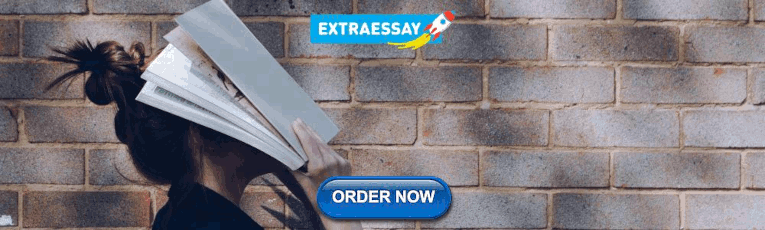
Plant People
Apart from genre fiction, human identification with or as plants relegates characters to the textual margins in the modern literary canon, but that has not always been the case. Whether as gatherers and gardeners, farmers and gleaners, nomads and Natives, folks who raise and prepare plants to provide basic necessities like food, shelter, clothing, and medicine largely have done so at the edges of the canon. Yet how humans relate to plants appears to be guided by particular themes and locations in their literary representation.
A “notoriously enigmatic” staple of medieval European church décor, the “green man” carvings—typically a head fantastically entwined in, sometimes even emanating, greenery—have no corresponding representation in texts prior to or during their creation, leaving them open to interpretation as violent or menacing. 26 But, in pointed contrast to plant-horror’s tendency to depict plants as a mass menace, the foliate head usually appears in singular form, and so might be seen instead as a link to ancient literary representations of plants as motifs of personhood, kinship, and subjectivity. 27 BIPOC and feminist literary critics demonstrate how traditional ecological knowledges hinge on attending to plants as people in ancient stories. Paula Gunn Allen’s The Sacred Hoop ( 1986 ) shows how translating human-plant in terms of strictly human relations warps Native American storytelling traditions of the “yellow woman” central to her Keres culture; representing their main grain maize, yellow woman’s stories instill agricultural knowledge together with the traditionally gynocentric values of Keres people. Whether understood to be a staple of folklore in ancient cultures or a folklorist invention of modern paganism, the green man’s stories, like those of the yellow woman, foster a sense of wonder at the seasonal rebirth characteristic of deciduous trees and other nonevergreen plants, inviting comparison with fictional tricksters likewise associated with greenery. In UK literary traditions, Sir Gawain’s green-skinned Green Knight, Sherwood Forest’s green-clad bandit Robin Hood, even Kensington Gardens’s Peter Pan all trouble figures of authority, both by trespassing on lands marked as royal and by asserting pre-Christian ideologies.
Various interpretations open to the titular character of Kingsley Amis’s novel The Green Man ( 1969 )—a “tree monster” / “tree devil,” a psychological projection of the main character, or just the pub of the same name that figures prominently in the story—add to the sense that shared plant-human being appears deeply unsettling in modernity, even in fantastical settings. Contemporary sf writers in the United States invoke this figure still more directly to develop the narrative potentials of human-plant reciprocity for social and environmental critique. Both Robert Jordan’s series The Wheel of Time ( 1990–2013 ) and Gene Wolfe’s series The Book of the New Sun ( 1980–1983 ) explicitly label as “the green man” an individual character who has special powers to manipulate plant life. In Ursula LeGuin’s The Word for World Is Forest ( 1972 ), decolonial becomes also ecological justice when the Athsheans, green-furred hominids who evolved in symbiosis with their forests, rise up against their enslavement by Earthlings and other aliens who disparage them as “little green men,” and effectively halt the invaders’ planetary-scale deforestation. 28
Comics paint in broader strokes how ecoconsciousness is being advanced by such characters. The 1963 introduction of the character Plantman, who stimulates plants to do his evil bidding, in Strange Tales and later X-Men lofts a dark narrative of Cold War science, combining plants, men, and chemicals that gives way to greener visions within the ensuing decade. Later comic representations of especially plant hybridity extend instead the ecological sensibility popularized by Rachel Carson’s bestselling Silent Spring ( 1962 ). The two most successful examples of ecological comics, Swamp Thing and Man-Thing , were first issued in 1972 and feature plant-human hybrids evolving through their respective narratives to become stewards of their swamps, even of “all realities” in the case of Man-Thing . What makes these new green men stories pivotal is their positioning of the human as a connective node within (not, like Plantman, a manipulator above and beyond) highly contingent multispecies communities, namely the microcommunities of their swamps. Perhaps most indicative of evolving eco-consciousness are the bad-to-good transformations of two more characters: Poison Ivy, introduced in 1966 as a supervillain botanist and biochemist, and the Plant Master (later Floronic Man), introduced in 1962 as a Plantman-like botanical manipulator, except also an alien exiled from an interdimensional world called Floria. Each was initially conceived as instrumentally using plants or plant qualities. Pivotally drawn together with Swamp Thing in Black Orchid ( 1989 ), they become repositioned to be recast in ensuing stories, respectively, as eco-warrior antiheroine and straightforward superhero.
The different but entangled trajectories of Poison Ivy and Floronic Man also show how conflicted relations proceed from differently gendered representations of shared human-plant embodiment. Ovid’s Metamorphoses (8 ce ) casts a long shadow over transformations of especially women and girls into flowering plants and trees in Western literary traditions. The story of Baucis and Philemon—who show hospitality to strangers later revealed to be gods in disguise, and therefore are rewarded by deathbed transformation to intertwining oak and linden trees—is referenced and repeated faithfully by the likes of Shakespeare, Hawthorne, and Powers along with Jean de la Fontaine, Jonathan Swift, Nikolai Gogol, Andre Breton, and Max Frisch to idealize heterosexual coupling. Not so is Daphne’s more abrupt change into a laurel to be saved from impending rape by Apollo, who then crowns himself with her wood; it is the rare literary reboot like Will Boast’s Daphne ( 2018 ) that resists her reduction to the vegetal/female object used by the animal/male superior force. 29 More typical are interpretations, for instance, of the title character of The Vegetarian as becoming silent, sessile, and otherwise plant-like by the end of the story, through the course of which she has already been depicted as brutally attacked, drugged, and ultimately institutionalized, indicating how the dangers of becoming plant-identified persist for some kinds of people.
The risks appear mitigated for those presenting as males voicing their own vegetal transformations. Walt Whitman’s willing himself to the reincarnating “grass of graves” is celebrated as one of the poet’s many gestures toward radical democracy in Leaves of Grass ( 1855 ). In Kobo Abe’s Kangaru Noto ( 1977 , translated as Kangaroo Notebook , 1991 ), the protagonist’s discovery of radish sprouts growing on his legs initiates a surreal medical journey to what he recognizes as Hell. Reflecting Abe’s formative years as a settler in Japanese colonial Manchuria and crediting the influence of Lewis Carroll, the novel meanders surrealistically, through “different things, creatures, and space-times, dreamings and stories.” 30 Only his story takes a uniquely weird turn along the way as he discovers that snacking on them makes him no longer able to stomach any sprouts except those grown on his own body. His growing sense of mutualism with his sprouts provides a tenuous connection back to another dimension of Ovid’s many tree-people, namely, their sense of more long-lived plants as benevolent personalities. It also provides the pretext for Le Guin’s “Olders” ( 1996 ), another transformation tale of a man, in this case in a coma, changing into the tree form in which he will join the grove of his likewise life-extended ancestors.
Trees for the Forest
“I think that I shall never see / A poem lovely as a tree” begins the most famous work by Joyce Kilmer, and it concludes: “Poems are made by fools like me / But only God can make a tree.” A strangely monotheist rewriting of the dendrolatry or tree-worship common to many peoples (including Kilmer’s Celtic ancestors), the poem’s framing of the tree exclusively as an aesthetic inspiration contrasts its roots in sacred texts. 31 Whether anchoring concepts of life, knowledge, or immortality, particular trees are central to folklore, mythology, and religion—the Biblical tree of the knowledge of good and evil, the Bodhi Tree or Buddha’s tree of awakening, and again Yggdrasil, the cosmically central Old Norse world tree from whom the god Odin hangs himself. Passionate paeans to trees also can be found in the works of William Blake, Rabindranath Tagore, Robert Frost, Pablo Neruda, Robert Macfarlane, and Mary Oliver—so prevalent, indeed, across cultures and periods that a comprehensive list might be endless—but individual connections like Odin’s prove more portentous.
In feminist fiction, the wavering of greenery between forefront and background roles can be traced in between two of Charlotte Perkins Gillman’s stories. “The Giant Wisteria” ( 1891 ), under which is uncovered the secret grave of a daughter murdered by her mother for having an illegitimate child, seems complicitous in the cover-up, if not an active menace. Whereas, in “The Yellow Wallpaper” ( 1892 ), the heroine narrating the spectacular backfire of bedrest to cure her mental illness ultimately envisions herself literally fading into the vegetal design papering the room. An inverse trajectory—from décor to live trees—unfolds within The Vegetarian , in the subplot of an artist obsessed with painting and filming floral designs on skin, who has an affair with his model Yeong-hye, who is also his sister-in-law and the character who becomes vegetative through the course of the story. Tellingly, after accidentally viewing a film of them having sex, the betrayed wife/sister In-hye ends the novel by staring at actual trees, “as if for an answer.” 32
Trees take on still more special roles as witnesses to atrocities. In representations of the US history of lynching, individual trees loom ominously. While Billie Holiday’s 1939 sound recording powerfully popularized the metaphorical rendering of “black bodies swinging in the southern breeze” in Abel Meeropol’s 1937 song “Strange Fruit,” literary writers of preceding decades imagined the trees themselves as narrators or witnesses. Paul Lawrence Dunbar’s “The Haunted Oak” ( 1913 ) describes a lynching from the perspective of a tree, recounting the story of a man hung by a mob from its bough. 33 A generation later, Richard Wright’s “Between the World and Me” ( 1935 ) reduces the tree to “a charred stump of a sapling,” though likewise capable of responding to the injustice only silently, by “pointing a blunt finger accusingly at the sky.”
Contemporary fictional representations of trees as living testaments to lynching include Jesmyn Ward’s Sing the Unburied, Sing ( 2017 ), in which the arboreal witness is imagined as a gathering place for ghosts of African American victims of many more racially motivated killings, too. The spectacle of Native Americans murdered by hanging haunts the fateful cottonwood grove of Leslie Marmon Silko’s novel Almanac of the Dead ( 1991 ). Louise Erdrich’s The Plague of Doves ( 2008 ) imagines as well the transgenerational trauma following a particular historical incident in which three Native Americans—including one child—were hung by white-settler vigilantes, showing how the tree becomes not just an instrument of racialized violence but also ultimately a gathering place for the victims’ surviving tribespeople, alongside the ghosts of passenger pigeons, by then driven to extinction by the same killers. An early example also based on a true story, Annette von Droste-Hülshoff’s 1844 murder mystery Die Judenbusch ( The Jew’s Beech ) uses the supernatural more forcefully to bring to justice the killer Friedrich, who by the end is found hanging from the tree under which his victim Aaron was found, and subsequently into the bark of which Friedrich’s fate cryptically had already been carved in Hebrew.
The idea that individual trees become mediators of social and personal tragedies is prevalent in many more contemporary novels that cast them realistically as significant presences. A historic tree, the Splittereiche (Splinter Oak) that survived the traumatic February 13–14, 1945 bombing of Dresden, serves in Marcel Beyer’s Kaltenberg ( 2012 ) as both living witness and connection to the narrator’s parents, who were lost that night; unlike the mass graves where they may be buried, visiting the tree enables him to conjure up memories of them. 34 Identifying unique potentials for postcolonial critique, Wendy Woodward compares representations of individual “arboreal beings” in several southern African fictions, including Zakes Mda’s The Heart of Redness ( 2000 ), Niq Mhlongo’s title short story in his collection Soweto, under the Apricot Tree ( 2018 ), and Mia Couto’s Under the Frangipani ( 2001 ), to show how they “foreground the presence of trees in their narratives, all implicitly critiquing a ‘culture’ which neglects to acknowledge a tree in its full significance.” 35 Her further relation of these individuals to a grove that serves a similar function in Beverley Rycroft’s A Slim Green Silence ( 2015 ) suggests an even greater literary force for trees represented en masse. Just as they provide the material backbone of so many vegetal communities, trees appear to be the most dwelt-upon botanical subject in literature, if not always appreciated as such.
Forest for the Trees
“A culture is no better than its woods” is the concluding line to W. H. Auden’s poem “Buccolics, II, Woods (for Nicholas Nabokov)” ( 1955 ), which begins “Sylvan meant savage in those primal woods.” If Robert Pogue Harrison’s Forests: The Shadow of Civilization ( 1992 ) establishes that as far back as Gilgamesh “forests represent the quintessence of what lies beyond the walls of the city,” then Auden’s poem exposes how they come to serve as a modern poetic measure of humanity’s failure to prove ourselves civilized. 36
Forests have long served as transformative zones in literary traditions of cultures rooted in arboreal regions of the world, whether as sites for carnivalesque inversion, like Robin Hood’s Sherwood Forest, or the unsolvable murder mystery played out among the bamboo of Ryunosuke Akutagawa’s “In a Grove” ( 1922 ), a story best known outside Japan through the classic film adaptation Rashomon ( 1950 ). Shakespeare’s A Midsummer Night’s Dream ( 1596 ), Ann Radcliffe’s Romance of the Forest ( 1791 ), all the versions before and since of the story captured in the Brothers Grimm’s “Little Red Riding Hood” ( 1812 ), even Stephen King’s The Girl Who Loved Tom Gordon ( 1999 ) position forests as places of intrigue and, especially for women and girls, danger. The trope of the deep, dark woods—whether as an uncanny place for humans, the home of monsters, or itself an active threat—has deep roots in specific, purportedly haunted woods, a staple especially of the English countryside, and consequently has strong attraction for eco-gothic writers today. 37
Literary history identifies more nuanced roles played by forests in particular periods and cultures. Albrecht Classen’s The Forest in Medieval German Literature ( 2015 ) examines canonical texts like the Nibelungenlied ( c . 1200 ) alongside lesser-known poetry and prose to complicate perceptions of forests as uniformly inhospitable, let alone as horror-filled, by showing how they guide characters’ development and renewal. Jeffrey Theis’s Writing the Forest in Early Modern England ( 2009 ) likewise reads Shakespeare, Andrew Marvell, and John Milton together with literature of the revolution like James Howell’s Dendrologia, Dordona’s Grave, or the Vocall Forest ( 1640 ) to show how literary and other writers expressed early concerns about forest law and deforestation. Citing Ojibwe traditional knowledges along with William Bradford, James Fenimore Cooper, John Muir, and Jim Harrison, among others, John Knott’s Imagining the Forest: Narratives of Michigan and the Upper Midwest ( 2012 ) uses literary and other stories of one North American region from the colonial period to the present to chart the emergence of two competing metaphors of the forest: as antithetical to civilization, in need of subjugation; and as cathedral, to be revered and protected.
More deeply mixing these impulses, a minor literary tradition imagines communities of arboreal beings as figures for social satire and eventually ecocriticism. Ludvig Holberg’s Nicolai Klimii Iter Subterraneum ( Niels Klim’s Underground Travels , 1741 ) imagines animate and articulate trees explaining their utopian world to a human visitor and suggests how literary representations of forests ground profound critiques within European Enlightenment thinking. These elements recur across the literary history of sf, most prominently perhaps with J. R. R. Tolkien’s old, wise, and otherwise treelike Ents of the Lord of the Rings series ( 1954–1955 ). 38 Similar creatures include Edgar Rice Burroughs’s otherworldly Plant Men of Barsoom in his Martian fiction series ( 1912–1943 ), as well as the sentient humanoid-cactus-like Cactacae of China Miéville’s Bas-Lag series ( 2000–2005 ).
The poor treatment of plants by people is a common complaint among literary treelike beings, though largely voiced from the sidelines. Much rarer are literary works narrated from the perspective of a tree like LeGuin’s “The Direction of the Road” ( 1974 ), in this case a stately oak who disdains apples in orchards as domesticated and thereby cognitively diminished “herd trees.” While casting doubt on the human character Olivia’s claim to hear individual trees speaking to her, Powers’s The Overstory ( 2018 ) further explodes this perspective on a global scale, imagining other humans tuning in less directly to arboreal chemical conversations circulating worldwide. Updating readers on the latest scientific findings as well as the cascading social and environmental consequences of replacing old-growth forests with tree plantations for capital gain, The Overstory as a whole complicates the picture through a self-reflexive critique of literature itself as obscuring the ethics of human-plant relations. Whether as e-books or print-copy “tree books,” bestsellers like The Overstory represent large-scale consumptions of forest products and other environmental threats, raising questions about how even sympathetic literary authors contribute to the slide of uses into abuses of plant life.
With vast monocrop plantings as a backdrop, literature primarily records the human toll of the intensification of agriculture made possible through settler colonialism, which not only has led to widespread diminishment and extinction of wild plant species but also to the movement and cultivation of plant species worldwide for the profit of few and through the victimization of many. Set on a sugar and cotton plantation in the southern United States, Harriet Beecher Stowe’s anti-abolitionist novel Uncle Tom’s Cabin: Or, Life among the Lowly ( 1852 ) has long been touted as an example of literature’s power to enact social change, a legacy overshadowed by its trade in poisonous stereotypes of black people. 39 Attending to the intertwined diminishment of human and nonhuman lives moves critics more generally to reframe the Anthropocene as the “Plantationocene” era, indicating how, even amid critiques of exploitation, hope can take root in literary representations of humans tending to continuities with plants.
Although the dramas of animal husbandry dominate the farm novel tradition, plants gain greater significance in examples with more direct historical grounding in the imperiled rights of horticulturalists. Dīnabandhu Mitra’s influential play Nil Darpan ( The Indigo Mirror , 1860 ) recounts a revolt that began with poor Bengali farmers refusing to plant indigo under exploitative conditions imposed by British Raj planters; as the first play staged by the National Theater in Kolkata, it is credited with advancing decolonial politics. At many levels, Charles Chesnutt’s short story “The Goophered Grapevine” ( 1899 ) identifies African American slaves and freemen with the titular vine, all together victimized by the greed of plantation owners and entrepreneurial northerners who replace them. Mixed reception histories call attention to how peoples’ phytophilia or attachments to plants can complicate the indigenous/invasive hostilities long attributed to texts like Isak Dinesen (Karen Blixen)’s Out of Africa ( 1937 ), a white woman’s memoir about starting and losing a coffee plantation in what later became Kenya. 40 In the US, decolonial politics take a backseat through novels like Frank Norris’s The Octopus ( 1901 ) and The Pit ( 1903 )—the first two books of his unfinished Epic of Wheat trilogy—along with John Steinbeck’s The Grapes of Wrath ( 1939 ) and John Nicholls’s The Milagro Beanfield War ( 1974 ), all of which concern the dangers of corporate interests to local smallholders who grow, harvest, and market crop plants.
Scientific and technological developments to optimize plant propagation inspire fantastic literary satire. Projecting a nightmare scenario of the Green Revolution avant la lettre , Ward Moore’s sf classic Greener Than You Think ( 1947 ) focuses on a suburban Californian lawn that, after mistakenly being treated with a chemical fertilizer that enables plants to turn anything into nitrogen, takes over the world. An imaginative departure from flesh-eating plant horrors, Moore’s grass makes people feel alienated from their own planet, confounds morphological resemblances, and ultimately overwhelms anthropocentrist fantasies. 41 Albeit in contemporary realist style, Ruth Ozeki’s All Over Creation ( 2003 ) tells a similar tale of plant alteration through science leading to unintended, global-scale changes. Shadowing the true story of how genetically modified potatoes became the first gene-altered plant to be widely grown for and then withdrawn from commercial markets, Ozeki draws different people’s stories—including those of farmers, organic activists, fast food workers, public relations specialists, and, perhaps most importantly, teachers—together with stories of potatoes’ multifarious reproductive and cross-cultural significances. Unlike Moore’s story, Ozeki’s ends with a sense of human-plant solidarity. For, nestled within the biodiversity desert of its Midwestern US monocrop-farmland setting is a green oasis: an elderly Japanese war bride’s wildly chaotic garden plot, filled with an incredible mix of vegetables, flowers, and more, which serves as a stage for reconciling fractured friendships and family relations amid the fight against corporate takeovers. 42
Garden, Greenhouse, and Potted Plants
Gardens function to bring people together for nourishment and healing, but not evenly, in life as in literature. Edenic qualities attributed to flower gardens in English country-house poems like Marvell’s “Upon Appleton House” ( 1651 ) naturalize the aristocratic status of the patrons whose praises they sing. Such associations persist in Frances Hodgson Burnett’s The Secret Garden ( 1910 ), where sickly children of the rich unlock what becomes for them the titular paradise, but only after they get a servant boy to restore it to order. Burnett herself fits a pattern of modern writers who draw inspiration from their own experiences of directing staff to build and manage extravagant country manor house gardens, alongside Beverley Nichols, the author of garden-writing hits like Down the Garden Path ( 1932 ), whose effete horticultural enthusiasms in turn prove ripe for immediate lampooning in W. C. Sellar and R. W. Yeatman’s Garden Rubbish and Other Country Bumps ( 1936 ). Literary representations of grand-scale gardening all the more clearly devolve into signs of losing the common touch amid landscapes transformed by widespread suffering.
With Vita Sackville-West’s grand gardens at Sissinghurst Castle long since transformed into a popular UK tourist attraction, her musing in “The Garden” ( 1946 ), “Small pleasures must correct great tragedies, / Therefore of gardens in the midst of war / I boldly tell,” seems worlds away from the more practical Victory Gardens promoted in plays of the time like Carey Miller’s Hoe! Hoe! Vitamin ( 1944 ), written as a practical intervention into the wartime starvation and malnutrition of the British populace. 43 That said, nostalgia persists for a sense that plant-tending provides a respite from the world, whether literally a safe space away from the hoi polloi in the case of Jerzy Kocinzki’s Chance, the idiot-savant gardener in Being There ( 1970 ), or a psychological respite from threats of murder and mayhem for the title character in The Constant Gardener , the bestselling 2001 John Le Carré novel and feature film based on a true story.
As Ozeki’s novel indicates, gardens are not just refuges from social struggles but also places for securing intimate ties amid them. Rosemary Manning’s The Chinese Garden ( 1962 ) features characters discovering a secreted and transformative space at a girls’ boarding school, only there it becomes a place for coming to terms with lesbian desire. The titular plant of Shani Mootoo’s novel Cereus Blooms at Night ( 1996 ), a species of cactus colloquially known as the “pass-along plant,” is an exotic transplant to a Caribbean garden that connects an intergenerational, queer, and racially mixed network of people who share and transplant cuttings from it, one that anchors a self-sustaining sense of fleeting, paradisiacal, “roving queer island garden” spaces for those who are otherwise set to be crushed by “the postemancipation plantation machine of the West Indies.” 44 Tan Twan Eng’s historical novel The Garden of Evening Mists ( 2012 ) makes the creation and restoration of a formal Japanese garden an impetus for healing from the loss of loved ones to the Japanese occupation of Malaysia during World War II. Eng’s aesthetic logic is clarified by a curious historical footnote: Japanese follow Chinese garden designs by favoring particular plant species praised in Classical Chinese literature, the point being circularly to create places of contemplation that in turn inspire more literary and artistic production.
That plants’ medicinal properties are central to folkways around the world lends gardens persistent dramatic value. Lacking affordable health care in contemporary small-town Mississippi, the African American protagonists of Ward’s novel tend in their gardens the makings of herbal remedies and charms depicted as easing, even saving, their loved ones’ lives. Such characters have many counterparts in earlier US literature, including Sarah Orne Jewett’s Eurowhite Mrs. Todd and Linda Hogan’s Native American Aunt Moon, both introduced as quirky botanophiles but later revealed to be powerful members of communities dependent on their herbalist expertise. Proulx’s 2016 novel Barkskins offers ironic commentary on this pattern through the character Sapotista, the great-great-great-great granddaughter of Mi’kmaq Mari, who was famed in the contact era for her North Woods herbal expertise. Uneasily inheriting Mari’s legacy, Sapotista herself earns a PhD in botany and exhorts students to turn their focus from “the old medicine plants [that] grew in a different world” before the forests were destroyed, in order to work as restoration ecologists to try to create conditions in which forest plants can regain their healing powers in the 20th century . 45
That such characters are predominantly women has roots in the practical needs of female embodiment, according to historian Londa Schiebinger, who points to works by Giovanni Boccacio, Ben Jonson, and Mary Wollstonecraft to show how “popular imaginative literature sheds light on early modern women’s use of abortifacients” like savin. 46 Genre fictions make much of associations of herbalism more explicitly with the dark arts, hence herbology featuring as a major discipline of study at J. K. Rowling’s Hogwarts School of Witchcraft and Wizardry across the Harry Potter series of novels and films. The predominance of female poisoners in murder mysteries—notably those of Agatha Christie, who herself trained as an herbalist before becoming a writer—directly speaks to a period in which the professionalization of pharmacology was leveraged by men’s relentless discrediting of female herbologists. 47
Given the preponderance of female authors and characters in literary representations of herbalism, it is notable that so many more male authors and characters are linked together as plant-based drug users and abusers. Debates continue about whether and how literary depictions glamorize or critique psychoactive experiences with derivatives of poppies, coca, marijuana, and more. Stories as diverse as Thomas De Quincey’s Confessions of an English Opium-Eater ( 1821 ), M. Ageyev (Mark Levi)’s Roman s Kokainum ( Novel with Cocaine , 1934 ), Ralph Ellison’s Invisible Man ( 1952 ), Irvine Welch’s Trainspotting ( 1993 ), and Marlon James’s A Brief History of Seven Killings ( 2014 ) become controversial also amid the unevenly experienced dangers of illicit drug trade for people of different classes, ethnicities, races, and nationalities. T. C. Boyle’s Budding Prospects: A Pastoral ( 1984 ) is an outlier in this tradition for its depiction of a young man’s drug use leading to his involvement in cultivation of a marijuana farm, and with a happy ending at that.
The introduction of greenhouses highlights how literature teems with the dramas of human and other animal lives, among which botanical subjects devolve to proverbial wallflowers in lockstep with their colonial exploitation for commercial markets. The faddish florist flowers and parlor flower stands heralding the Georgian era come to the fore at the end of Jane Austen’s last novel Persuasion ( 1818 ), when the star-crossed protagonists’ mutual “admir[ation of] a fine display of greenhouse plants” provides a ruse for rekindling their love affair. 48 Victorian lovers gain a secreted space of luxury by escaping to the home conservatory, an architectural novelty that is itself a sign of conspicuous consumption in Charlotte Yongue’s novel The Daisy Chain, or Aspirations: A Family Chronicle ( 1856 ). 49 Fueling a vogue for greenhouses open to the eventually wearying public, the scene of literary hothouse love devolves to a cliché by the Edwardian era, as illustrated by an assignation at “the usual palm tree,” or “the second palm tree on the left” in Oscar Wilde’s play An Ideal Husband ( 1895 ). 50 Raymond Chandler’s iconic noir novel The Big Sleep ( 1939 ) flags the monstrous turn in the 20th century . An early scene features Philip Marlowe’s meeting in the greenhouse with General Sternwood, whose name along with his description as a “bloodless,” cold-sensitive, paraplegic, old, dying man aligns with his cramped plants, ominously described as “a forest of them, with nasty meaty leaves and stalks like the newly washed fingers of dead men.” But even Sternwood hates them, particularly the orchids, declaring: “They are nasty things. Their flesh is too much like the flesh of men. And their perfume has the rotten sweetness of a prostitute.” Instrumentally or decoratively filling in the scenery of human dramas, the literary destiny of such vegetal presences verges from unsympathetic to detested.
No living beings populate literary history more inconspicuously than houseplants, yet their growing presence in 20th-century contexts appears distinctly to their detriment. Identifying with a potted plant signals that a character is pathetic, sympathizing with its sufferings perverse, and truly caring for them pathological. Far from the hothouse and parlor displays, lonesome Fanny in Austen’s Mansfield Park ( 1814 ) is promised a puppy and sometimes lent a horse but ultimately makes daily life in her cold rooms bearable by bringing in potted plants. By the 20th century , fictions feature self-isolated and privileged white men who, although they claim to care about plants, only act to torture, kill, or even just leave plants unpotted to die of root exposure. The protagonists of George Orwell’s Keep the Aspidistra Flying ( 1936 ), Flannery O’Connor’s “The Geranium” ( 1946 ), and Mary McCarthy’s Birds of America ( 1965 ) all fit this bill.
Twenty-first-century examples suggest that this pattern might be changing, as the sense of growing relations between people and particular houseplants indicates how they can provide a basis for reconceptualizing difference or otherness. Extremely socially isolated and abused characters are identified with plants only in ways that highlight systemic social problems in Chimamanda Adichie’s Americanah ( 2014 ) and Haruki Murakami’s Ichi-Kyu-Hachi-Yon ( 2009–2010 ), translated as 1Q84 ( 2011 ). Both stories explicitly address the spread of and limits to settler cultures, respectively, in Africa and Asia, suggesting how enlisting potted plants in these investigations can open new avenues for social modeling and critique beyond Euro-American traditions. 51
Botanical Memoirs
Naturalist writing on forests tracks growing ecological sensibilities, and bleeds into botanical memoir amid the fraught histories of local peoples’ fights for environmental justice. While plant writing peppers all the work of Henry Thoreau, The Maine Woods ( 1864 ) most clearly articulates a vision of living off forested land, even while expressing sympathy with the peoples whose lifeways and lands alike were even then obviously being endangered by commercial interests, particularly loggers. (Telescoped across the Great North Woods into Canada, a similar but fictional story snowballs across generations, continents, and cultural perspectives in Barkskins .) The intersections of genres also prove useful for articulating the imbrication of scientific and literary writing about plants. Known mostly for his popular bird books, biologist Bernd Heinrich revisits a slice of the Maine woods in a likewise lyrical memoir, The Trees in My Forest ( 1998 ), to share his sense and sensibilities of arboreal being, and to detail recent scientific findings that affirm them.
Although not memoirs per se, botanical writing by authors with extensive experience working with plants, often as scientists, enjoys enduring popularity. Recognized more for her skills in plant propagation than her poetry in her lifetime, Emily Dickinson’s writing is filled with references to species that she cultivated in her gardens, meadow, and conservatory, many of which are preserved in a herbarium that she created as a schoolgirl. 52 In the 21st century , forester Peter Wohlleben’s runaway bestseller Das geheime Leben der Bäume: Was sie fühlen, wie sie kommunizieren; Die Entdeckung einer verborgenen Welt ( The Hidden Life of Trees: What They Feel, How They Communicate; Discoveries from a Secret World , 2015 ) indicates public receptivity to perceptions of trees as social creatures whose needs intersect with those of humans. In Powers’s The Overstory , the fictional dendrologist Patricia Westerford’s similarly sensational The Secret Forest , which is the lone point of connection across the sprawling novel’s diverse cast, appears to have been modeled after the life of biologist Susanne Simard. Life imitating art, Simard’s own Finding the Mother Tree: Discovering the Wisdom of the Forest ( 2021 ) is a story that blends personal and scientific discovery, more like biologist David George Haskell’s The Forest Unseen: A Year’s Watch in Nature ( 2012 ) and The Songs of Trees: Stories of Nature’s Great Connectors ( 2017 ) in weaving together autobiographical and nature writing for the purpose of advancing understanding of plants as communicative and social creatures.
A key intertext in Ozeki’s novel, The Harvest of the Years ( 1927 )—the autobiography of plant developer Luther Burbank, breeder of the world’s most-planted potato, the Burbank russet, among other popular cultivars—is an early example of a hybrid science- and life-writing genre that nearly a century later brings popular audiences to important critiques of science as well. Paleobotanist Hope Jahren’s Lab Girl: A Story of Trees, Science, and Love ( 2016 ) details the grant-writing mill, laboratory turf wars, and institution-hopping expectations that condition plant science in her experience as a successful academic, with special attention to the sexism she endured. Similarly science-skeptical autobiographies of descendants of enslaved and colonized peoples instead focus on field work to suggest that intentionally living and working with live plants provides a bulwark against cultural and biological endangerment alike. Focusing on the fragile dependencies on native plant species helps Robin Wall Kimmerer to articulate her journey to becoming an ethnobotanist and an engaged citizen of the Potowatomi Nation in Gathering Moss: A Natural and Cultural History of Mosses ( 2003 ) and Braiding Sweetgrass: Indigenous Wisdom, Scientific Knowledge, and the Teachings of Plants ( 2014 ). Though an ornithologist like Heinrich, J. Drew Lanham details in The Home Place: A Colored Man’s Love Affair with Nature ( 2016 ) the fruits and vegetables of his parents’ truck garden, the borderland battles of weeds and flowers in his grandmother’s garden, even the endangered wild plants native to the home of this African American family, all of which provide a sense of connectedness that proves crucial here, too, to the successful career of a BIPOC natural scientist.
At the turn of the 21st century , creative writers experiment with plants to develop critical reflections on themselves as well. Adding a new dimension to his reputation as a poet and journalist, James Fenton harnesses his hobbyist experience with garden economy in A Garden from a Hundred Packets of Seed ( 2001 ), which organizes by garden plant varieties a thought experiment in what to grow if limited just to seed. Sumana Roy’s likewise formally experimental How I Became a Tree ( 2017 ) mixes memoir, literary history, spiritual philosophies, and botanical research to explore how trees inspire her to pursue more satisfying ways of being human. Poets Ross Gay and Aimee Nezhukumatathil’s collaboratively authored Lace and Pyrite ( 2014 ) began as a commitment to weekly epistolary exchanges of poems over the course of a year, and the thematic centering on their respective obsessions with cultivating food and flowers is reflected in the title of an early excerpt, “Letters from Two Gardens” ( 2012 ). More recently Gay’s The Book of Delights , a genre-defying year in the life as told through short, daily reflections on something delightful, clarify further how an African American man finds much-needed pleasure in plants and plant writing amid the ongoing terrors highlighted by the Black Lives Matter movement.
Experimental life writing about plants is an emerging genre with enormous potential for enriching the broader efforts of decolonizing and restorative justice projects as well. Jamaica Kincaid reflects in her experimental autobiography My Garden (Book): ( 1999 ) on how her gardening practices weave together various relationships with plants that are personal, historical, and transformative. Building on the family-gardening-memoir elements of My Brother ( 1997 ), a story ostensibly about her sibling’s AIDS-complicated death, Kincaid explains how she began gardening after already becoming a popularly and critically acclaimed writer of the black diaspora only to find among plants a different content and form for contesting the limits of authorial identity, for refusing the master narratives imposed on “me and all who look like me,” in Kincaid’s phrasing. 53 A project that began as a mixed-race adoptee’s search for her own roots, Catherine McKinley’s Indigo: In Search of the Color that Seduced the World ( 2011 ) more deliberately digs into the histories of peoples and plant species along with plant knowledges similarly to question how they became endangered or lost in the African diaspora, and why creatively adapting genres like autobiography are a necessary part of their recovery and restoration.
Discussion of the Literature
The tendency of literary scholars inspired by critical plant studies to highlight modern and contemporary literature suggests a tremendous opportunity to track plant thinking in older texts. The oldest examples of scholarship on plants and literature have emerged through classical and medieval studies of herbalism as a nexus of botanical, cultural, and medical knowledges. An early example that illustrates the importance of etymology to understanding ancient botany and medicine is Reginald Campbell Thompson’s The Assyrian Herbal: A Monograph on the Assyrian Vegetable Drugs . A century later, studies like Rebecca Armstrong’s Vergil’s Green Thoughts: Plants, Humans, and the Divine trace the porous boundaries of science and nature writing within the canon of classical studies by attending to their representations of “the contrast and intersection of rational and non-rational reactions to plants: the emotional and superstitious associations they evoke, together with scientific and pragmatic understandings of their properties.” 54 Attending not only to how texts were originally produced but also their subsequent disseminations, preservations, and sometimes distortions clarifies further the relevance of the history of plant writing to literary studies. Focused on a case in which “a once vital medical text was transformed into a literary curiosity,” Anne Van Arsdall’s Medieval Remedies: The Old English Herbarium and Anglo-Saxon Medicine uses translation history to clarify the adaptation of ancient Roman knowledge to British medieval contexts, resulting in immediate enrichments, yet also eventual diminutions, of these knowledges across Europe and the Americas. 55
Widening scholarly focus beyond Euro-American examples is a persistent challenge largely taken up by BIPOC scholars and creative writers. More ordinary representations, for instance, of planting watermelon seeds help Gloria Anzaldúa to pinpoint her own mestiza status in Borderlands/La Frontera: The New Mestiza , at once celebrating her queer and Anglo-Latinx-Native American hybridity and expressing concern for its fragility deep in the home of her farming family, Hidalgo County in Texas, which despite great agricultural and cultural richness perennially ranks among the economically poorest parts of the US. 56 In Alice Walker’s In Search of Our Mothers’ Gardens , gardening serves as a metaphor for her own “womanist” aesthetic (“with every color flower represented”) that, quite apart from any anxiety of influence, fosters appreciation for continuities across famous black women artists and writers along with her own mother’s lone aesthetic outlet, her flower garden. 57 In contrast, the customary inattention to the literary histories marginalizing especially BIPOC women’s perspectives compounds the ableist offensiveness affixed to writers’ “plant blindness” and attempts to remedy it alike in literary criticism.
In Plants and Literature: Critical Plant Studies , Randy Laist attributes the dearth of “plant-based narratives” to a broader “defoliation of the cultural imagination” that follows from the withering “botanical vocabulary” of urban-industrialized peoples. 58 The predominant approach in literary criticism instead has favored what John Charles Ryan, in Plants in Contemporary Poetry: Ecocriticism and the Botanical Imagination , terms “botanical criticism,” a tradition of interpreting representations of vegetal life only to marginalize any sense of plants as having their own lifeworlds. As an alternative, Ryan outlines more verdant potentials in what he terms “phytocriticism,” which “assesses the extent to which vegetal dynamism figures into the shape of cultural productions,” and emphasizes the importance of botanical agency for ecocriticism. 59 Patterns across poems interrogating perceptions of plants as bodies and souls, as signifiers of settings and agency, and ultimately as inspiration for melancholia and even hope gain special interest amid ever-increasing anthropogenic extinctions of untold species of flora along with the fauna dependent on them. However, Ryan’s application of his phytocritical approach exclusively to contemporary Anglophone poetry indicates that the question of “why now” may be easier to answer than “why look at literary plants in the first place.”
In the introduction to The Language of Plants: Science, Philosophy, Literature , Ryan together with Monica Gagliano and Patricía Viera calls for a more specifically “phytocentric” approach to literary criticism that “seriously would regard the lives of plants in relation to humankind in terms that would look beyond the purely symbolic or [objective-correlative] dimensions of the vegetal.” 60 However inadvertently, the handful of examples discussed in their volume’s “Literature” section indicate that such writing emerges sporadically, and only in post- 1800 white people’s writing. Joela Jacobs and Isabel Kranz’s bilingual coedited special issue of Literatur für Leser charts a broader range of histories and cultural perspectives that enrich their central concept of “botanical poetics.” 61 Though quite differently, these collections raise concerns about how genre, period, and cultural preferences create more obstacles than opportunities for studying plants and literature.
An earlier volume inspired by critical plant studies, Molly Mahood’s The Poet as Botanist samples a range of works by UK and US literary authors with formal scientific plant-related training and across the past 300 years. 62 Sharing Ryan’s focus on poetry, Mahood extends a literary-critical pattern set by Charlotte Otten’s Environ’d with Eternity: God, Poems, and Plants in Sixteenth and Seventeenth Century England . 63 Otten looks back still further to canonical early modern writers to trace how languages of flowers more specifically infuse theology with herbalism in British literature. That all three monographs separate and elevate botanical poetry in English over any other type of literature begs questions about how other literary forms and cultural traditions support still more plant-specific approaches, and for what purposes. 64
Without mentioning critical plant studies, several 21st-century volumes concentrate on Linnaean-influenced writers who muddy the science-literature divide and highlight linkages of experimental botanical writing and feminist critique. Elaine Miller takes a philosophical approach to Goethe’s poetry in The Vegetative Soul: From Philosophy of Nature to Subjectivity in the Feminine , making the case that it culminates in philosopher Luce Irigaray’s efflorescent interpretive writing practice, exemplifying “plant-like reading.” 65 Literary writers also find in plants inspiration to challenge the authority of analytic and experimental modes of inquiry in science, as again George’s Botany, Sexuality, and Women’s Writing, 1760–1830 likewise circles back to Erasmus Darwin in order to elaborate. Noting how the translation of Jean-Jacques Rousseau’s Letters on the Elements of Botany Addressed to a Lady ( 1785 ) was already “a surprise bestseller in England,” George explains how Darwin’s The Botanic Garden also inspired Wollstonecraft’s defense of botany against prudish reactions to Linnaean theories in her Vindication of the Rights of Women ( 1792 ), which immediately sparked numerous botanically themed satires. 66 Among the more lasting effects of the popular introduction of the Linnaean sexual system to the United Kingdom was the restriction of women’s access to botanical knowledges in favor of floriography—using flowers to send coded messages—which continues to be idealized through Vanessa Diffenbaugh’s bestselling 2011 chick-lit novel The Language of Flowers , published with a companion flower dictionary by Mandy Kirkby. 67 On the plus side, according to George, The Botanic Garden also led immediately to the innovation of “a new genre of women’s writing: the botanical poem with scientific notes.” 68 While most flowery-titled fictions today are nonbotanical romances, poet Molly Peacock’s experimental auto/biography The Paper Garden: An Artist Begins Her Life’s Work at 72 , which circles around her own fascination with 18th-century artist Mary Delaney’s massive, late-life production of hundreds of botanically correct, mixed-media collage representations of flowers (today housed as the Flora Delanica collection in the British Museum), provides yet another example of how women writers continue making formal innovations while directly struggling with these histories. 69
An enduring figure of the sublime, the blaue blume or “blue flower” introduced in Novalis (Friedrich von Hardenberg)’s unfinished novel Heinrich von Ofterdingen emerges in literary studies as the central symbol of German Romanticism. 70 But cross-cultural readings of the Romantic period further trouble a coherent plant politics in literature and literary criticism, whether ecocritical, feminist, or otherwise. Theresa Kelley’s Clandestine Marriage: Botany and Romantic Culture compares literary, scientific, philosophical, and visual art texts from the United Kingdom and its colonies in India and the Caribbean in order to highlight how Romantic plant representations undermine hierarchical thinking, throwing anthropocentric certainties into turmoil. Considering the highly variable spellings and wordings of common names for the same plant species in John Clare’s poetry, for instance, Kelley finds evidence of no eco-warrior but rather “a poet whose poetics of place, and especially of plants,” reacted against the doubled assault on place-based knowledge and language that was posed by Linnaeus’s systematization of standard plant names. 71 Her chapter on women’s plant writing of the period includes examples echoing Darwin, debating Goethe, and—notably in public disagreements between Wollstonecraft and similarly prominent, radical writer Anna Barbauld—conflicting with each other in their uncertain challenges to poisonous plantlike characterizations of femininity. Kelley’s approach complements earlier single-author studies that chart changing attitudes toward and interpretations of the significance of plants within the period, for instance, within John Keats’s oeuvre toward embracing floral imagery in ways that complicate received notions of sex and desire. 72
Intriguingly, a broader survey that cuts across cultures and periods further problematizes attempts to position literary writing as simply popularizing scientific or ethical understandings of plant life. Natania Meeker and Antónia Szabari’s Radical Botany: Plants and Speculative Fiction explicitly rejects Marder’s plant ethics as an imposition of human onto vegetal concerns, one that risks obscuring the important insight to which literary writing gives voice: how little we matter from plants’ perspectives. Charting convergences of botanical facts and fictions from the Enlightenment to the present, they make the case that select examples from literature, science, philosophy, and film constitute a minor tradition of “radical botany, in which plants are not just objects of manipulation but participants in the effort to imagine new worlds and to envision new futures.” 73 Compelled by Gilles Deleuze and Félix Guattari’s famous injunction to “follow the plants”—and consequently to model not only treelike hierarchies but also tuberlike “rhizomatic” or lateral structures—Meeker and Szabari are more openly hostile to ecocritical traditions that posit plants at best as handmaidens to Romanticist visions of nature as a mirror to the human mind, as well as to animal studies, which they see as perpetuating anthropocentric bias. 74 Meeker and Szabari instead locate the origins of radical botany in the writing of 17th-century French libertins érudits (libertine scholars). Fanciful philosophical fictions by Cyrano de Bergerac and botanical writings of contemporary Guy de La Brosse (most famous today for having created the royal medicinal herb garden that became Paris’s Jardin des Plantes ) tease out a common theme of representing plants as percipient and libidinal that cuts across French science fact and fiction at the outset and connects a broad range of subsequent Euro-American literary developments.
If, as Meeker and Szabari assert, “plants draw us in (in their strangeness) and exceed our social and ecological categories” only to “undo us as individuals and as part of an ecosystem,” then it seems necessary to ask: for whom are we writing how plant being becomes radicalized? 75
Christopher Columbus’s arrival in the Americas marks a frenzied era of plant movement around the globe as well as scientific knowledges and the development of systems to classify them, but less attention has been paid to how these developments also involve particular patterns in the representation of plant life, significantly including the deliberate curation of ignorance. 76 Reflecting on the same 400-years-and-more history—only foregrounding the colonial and postcolonial legacies of the enforced movements, renamings, erasures, and other violations of people alongside plants—Kincaid’s My Garden (Book): raises the question, what are nonwhite authors supposed to do with a story like this? An intriguing answer emerges through plant forms like Sargasso weed, a kind of seaweed that uniquely travels the routes and writings of the Black Atlantic as well as like much sea vegetation conceptually confounds tree, rhizome, and other land-based structures. 77 The disciplinary confusion and genre-melding aspects of botanical writing indicate that radicalizing literary forms along with poetics is part of an answer that remains much in need of further development.
Links to Digital Materials
- Botanical Speculations .
- Critical Plant Studies .
- Literary and Cultural Plant Studies Network .
- Critical Plant Studies: Philosophy, Literature, Culture .
Further Reading
- Aloi, Giovanni , ed. Why Look at Plants? The Botanical Emergence in Contemporary Art . Leiden, The Netherlands: Brill, 2019.
- Armstrong, Rebecca . Vergil’s Green Thoughts: Plants, Humans, and the Divine . Oxford: Oxford University Press, 2019.
- Butcher, Daisy , ed. Evil Roots: Killer Tales of the Botanical Gothic . London: British Library, 2019.
- Gagliano, Monica , John C. Ryan , and Patrícia Vieira , eds. The Language of Plants: Philosophy, Science, Literature . Minneapolis: University of Minnesota Press, 2017.
- George, Sam . Botany, Sexuality, and Women’s Writing, 1760–1830: From Modest Shoot to Forward Plant . Manchester, UK: Manchester University Press, 2007.
- Keetley, Dawn , and Angela Tenga , eds. Plant Horror: Approaches to the Monstrous Vegetal in Fiction and Film . London: Brill, 2016.
- Kelley, Theresa . Clandestine Marriage: Botany and Romantic Culture . Baltimore: Johns Hopkins University Press, 2012.
- Kincaid, Jamaica . My Garden (Book) : . New York: Farrar, Strauss, Giroux, 1999.
- Laist, Randy , ed. Plants and Literature: Critical Plant Studies . Leiden, The Netherlands: Brill, 2013.
- Mahood, Molly . The Poet as Botanist . Cambridge, UK: University of Cambridge Press, 2008.
- Marder, Michael . Plant-Thinking: A Philosophy of Vegetal Life . New York: Columbia University Press, 2013.
- Meeker, Natania , and Antónia Szabari . Radical Botany: Plants and Speculative Fiction . New York: Fordham University Press, 2019.
- Miller, Elaine . The Vegetative Soul: From Philosophy of Nature to Subjectivity in the Feminine . Albany, NY: State University of New York Press, 2002.
- Otten, Charlotte . Environ’d with Eternity: God, Poems, and Plants in Sixteenth and Seventeenth Century England . Lawrence, KS: Coronado Press, 1984.
- Parker, Elizabeth . The Forest and the EcoGothic: The Deep Dark Woods in the Popular Imagination . London: Palgrave, 2020.
- Ryan, John C. Forest Family: Australian Culture, Art, and Trees . Leiden, The Netherlands: Brill, 2018.
- Ryan, John C. Plants in Contemporary Poetry: Ecocriticism and the Botanical Imagination . New York: Routledge, 2018.
- Theis, Jeffrey . Writing the Forest in Early Modern England: A Sylvan Pastoral Nation . Pittsburgh, PA: Duquesne University Press, 2009.
- Van Arsdall, Anne . Medieval Remedies: The Old English Herbarium and Anglo-Saxon Medicine . New York: Routledge, 2002.
- Yates, Julian . Of Sheep, Oranges, and Yeast: A Multispecies Impression . Minneapolis: University of Minnesota Press, 2017.
1. Lawrence Buell, quoted in John C. Ryan, Plants in Contemporary Poetry: Ecocriticism and the Botanical Imagination (New York: Routledge, 2018), 7 ; and Erin James, “What the Plant Says: Plant Narrators in the Ecosocial Imaginary,” in The Language of Plants: Philosophy, Science, Literature , ed. Monica Gagliano, John C. Ryan, and Patrícia Vieira (Minneapolis: University of Minnesota Press, 2017), 270.
2. Michael Marder, “Plant Intentionality and the Phenomenological Framework of Plant Intelligence,” Plant Signaling and Behavior 7, no. 11 (2012): 1365–1372.
3. Michael Marder, book series description, Critical Plant Studies .
4. Molly Mahood, The Poet as Botanist (Cambridge, UK: University of Cambridge Press, 2008), 226–227 .
5. Matthew Battles, Tree (New York: Bloomsbury Academic, 2017), 73; and Elaine Miller, The Vegetative Soul: From Philosophy of Nature to Subjectivity in the Feminine (Albany, NY: State University of New York Press, 2002), 11–12 .
6. Stephen T. Jackson, “Introduction: Humboldt, Ecology, and the Cosmos,” in Essay on the Geography of Plants , ed. Alexander von Humboldt and Aimé Bonpland, trans. Sylvie Romanowski (Chicago: Chicago University Press, 2009), viii; and Laura Søvsø Thomasen, “Showing and Telling: The Integrated Use of Literature and Images in the Works of Erasmus Darwin and Alexander von Humboldt,” Interdisciplinary Science Reviews 42, no. 3 (2017): 27–240.
7. Londa Schiebinger, Nature’s Body: Gender in the Making of Modern Science (Boston: Beacon Press, 1993), 36–37.
8. Julian Yates, Of Sheep, Oranges, and Yeast: A Multispecies Impression (Minneapolis: University of Minnesota Press, 2017), 156 .
9. Patrícia Vieira, “Phytographia: Literature as Plant Writing,” Environmental Philosophy 12, no. 2 (2015): 208.
10. Vieira, “Phytographia,” 215. Vieira elaborates, “Plants endlessly repeat parts of themselves by producing multiple leaves, flowers and fruits, all sharing similar traits but also displaying minuscule differences. Vegetal life and inscription are thus eminently graphic and could be understood as the paradigmatic example of arche-writing.”
11. Vieira, “Phytographia,” 218. See also Vieira, “Phytofables: Tales of the Amazon,” Journal of Lusophone Studies 1, no. 2 (2016): 116–134.
12. See for instance the argument for the literary merits of 17th-century Dutch colonial naturalist writing like Rumfius (Georg Rumf)’s Amboinsch Kruid-boek ( Ambonese Herbal , 1741–1750) through comparison with US Romantic writers like Henry Thoreau in Eric Beekman, Troubled Pleasures: Dutch Colonial Literature from the East Indies, 1600–1950 (Oxford: Clarendon Press, 1996).
13. Joela Jacobs, “Phytopoetics: Upending the Passive Paradigm with Vegetal Violence and Eroticism,” Catalyst: Feminism, Theory, Technoscience 5, no. 2 (2019): 1–18.
14. Priscilla Wald, “Botanophobia: Fear of Plants in the Atomic Age,” Japanese Journal of American Studies 24 (2013): 7–27. See also Natania Meeker and Antónia Szabari, “From the Century of the Pods to the Century of the Plants: Plant Horror, Politics, and Vegetal Ontology,” Discourse 34, no. 1 (2012): 32–58.
15. Hsuan Keng, “Economic Plants of Ancient North China as Mentioned in Shih Ching ( Book of Poetry ),” Economic Botany 28, no. 4 (1974): 404–405.
16. Nathan Snaza, Animate Literacies: Literature, Affect, and the Politics of Humanism (Durham, NC: Duke University Press, 2019), 15–16.
17. Dhananjay Dwivedi, “The Importance of Plants as Depicted in Puranas ,” Indian Journal of History of Science 52, no. 3 (2017): 251–274.
18. Shakespeare, Richard II , ed. Anthony B. Dawson and Yachnin (Oxford: Oxford University Press, 2012); and Sarah Crover, “Gardening, Stewardship and Worn-Out Metaphors: Richard II and Justin Trudeau,” Early Modern Culture 13, no. 1 (2018): 152.
19. Rebecca Laroche and Jennifer Munroe, “On a Bank of Rue: Or Material Ecofeminist Inquiry and the Garden of Richard II,” Shakespeare Studies 42 (2014): 43.
20. Dawn Keetley, “Introduction: Six Theses on Plant Horror; Or, Why Are Plants So Horrifying,” in Plant Horror: The Monstrous Vegetal in Fiction and Film , ed. Dawn Keetley and Angela Tenga (London: Palgrave, 2016), 1.
21. Whitney Anne Trettien, “Plant -> Animal -> Book: Magnifying a Microhistory of Media Circuits,” Postmedieval 3, no. 1 (2012): 97.
22. “The Upas Tree,” Scientific American 13, no. 47 (1858): 374.
23. Daisy Butcher, “Introduction,” in Evil Roots: Killer Tales of the Botanical Gothic , ed. Daisy Butcher (London: British Library, 2019), 1.
24. Edmund Spencer, “Wonderful Stories: The Man-Eating Tree,” New York World , April 26, 1874.
25. Susan Orlean, The Orchid Thief: A True Story of Beauty and Obsession (New York: Random House, 2011), 74. See also Anne Goldgar, Tulipmania: Money, Honor, and Knowledge in the Dutch Golden Age (Chicago: University of Chicago Press, 2008), which corrects modern misperceptions of this phenomenon in part through references to the works of contemporary poets and pamphleteers.
26. Keetley, “Introduction,” 4.
27. Matthew Hall, Plants as Persons: A Philosophical Botany (Albany, NY: State University of New York Press, 2011), 126. Hall points to Elias Lönnrot’s 1835 epic poem Kalevala , which is drawn from Karelian and Finnish folklore and mythology, as exemplifying “the most complete and interesting European accounts of plant personhood,” including trees that give voice to their own self-awareness, 129.
28. Donna Haraway, “Sowing Worlds: A Seed Bag for Transforming with Earth Others,” in Beyond the Cyborg: Adventures with Donna Haraway , ed. Margaret Grebowicz and Helen Merrick (New York: Columbia University Press, 2013), 141.
29. Miller, The Vegetative Soul , 5.
30. Kaori Nagai, Imperial Beast Fables: Animals, Cosmopolitanism, and British Empire (New York: Palgrave, 2020), 148.
31. Manuel Lina, The Book of Trees: Visualizing Branches of Knowledge (Hudson, NY: Princeton Architectural Press, 2013), 6.
32. Han Kang, The Vegetarian , trans. Debora Smith (London: Hogarth Press, 2016), 181.
33. “The Direction of the Road” in Ursula Le Guin’s collection The Wind’s Twelve Quarters (New York: Harper and Row, 1974) is a rare short story for likewise being narrated from a tree’s perspective.
34. Solvejg Nitzke, “ Saxony’s Arboreal Curiosities, or: How to Root (Hi)Stories ,” Botanical Letters , last modified June 16, 2020.
35. Wendy Woodward, “Arboreal Being: Encounters with Trees in Recent Southern African Fiction,” Journal of Literary Studies 35, no. 4 (2019): 96.
36. Robert Harrison, Forests: The Shadow of Civilization (Chicago: University of Chicago Press, 1993), 17.
37. See Elizabeth Parker, The Forest and the EcoGothic: The Deep Dark Woods in the Popular Imagination (London: Palgrave, 2020) .
38. James Ignatius McNelis, “‘The Tree Took Me Up from the Ground and Carried Me Off’: A Source for Tolkien’s Ents in Ludvig Holberg’s Journey of Niels Klim to the World Underground ,” Tolkien Studies 3 (2006): 153.
39. Henry Louis Gates, Jr., “Kwame Anthony Appiah,” in Africana: Arts and Letters; An A-to-Z Reference of Writers, Musicians, and Artists of the African American Experience (Philadelphia, PA: Running Press, 2005), 544.
40. Peter Mortensen, “‘A Coffee-Plantation Is a Thing That Gets Hold of You and Does Not Let You Go’: Plant-Writing in Karen Blixen’s Out of Africa ,” Journal of Literary Studies 35, no. 4 (2019): 28–45. A generation later and from a child’s perspective, Elspeth Huxley’s The Flame Trees of Thika: Memories of an African Childhood (1957) is more critical of British paternalism toward African peoples and the senseless slaughter of wild animals, but likewise fails to question the settler monopoly on coffee growing in the region.
41. Vin Nardizi, “Greener,” in Prismatic Ecology: Ecotheory beyond Green , ed. Jeffrey Cohen (Minnesota: University of Minnesota Press, 2013), 155.
42. Susan McHugh, “Flora, Not Fauna: GM Culture and Agriculture,” Literature and Medicine 26, no. 1 (2007): 25–54.
43. See Char Miller, “In the Sweat of Our Brow: Citizenship in American Practice during WWII; Victory Gardens,” Journal of American Culture 26, no. 3 (2003): 395–409.
44. Jill Casid, Sowing Empire: Landscape and Colonization (Minneapolis: University of Minnesota Press, 2004), xx–xxi.
45. Annie Proulx, Barkskins (New York: Scribner, 2016), 696.
46. Londa Schiebinger, Plants and Empire: Colonial Bioprospecting in the Atlantic World (Cambridge, MA: Harvard University Press, 2007), 125.
47. Alicia Carroll “‘Leaves and Berries’: Agatha Christie and the Herbal Revival,” Green Letters 22, no. 1 (2018): 20–30. Carroll argues, “Reading to historicize plants, advocate for them, and locate their presence in literature, then, may uncover debates long forgotten and reveal the extent to which stereotypes like the female poisoner and toxic plants conceal a hidden history of the pains—and pleasures—of ecological interdependence.”
48. Deidre Lynch, “‘Young Ladies Are Delicate Plants’: Jane Austen and Greenhouse Romanticism,” ELH (English Literary History) 77, no. 3 (2010): 689–729.
49. Catherine Harwood, Potted History: The Story of Plants in the Home (London: Frances Lincoln, 2007), 123.
50. Oscar Wilde, Two Society Comedies: A Woman of No Importance and An Ideal Husband, ed. Ian Small and Russell Jackson (New York: W. W. Norton, 1983), 253.
51. Susan McHugh, “Houseplants as Fictional Subjects,” in Why Look at Plants? The Botanical Emergence in Contemporary Art , ed. Giovanni Aloi (Leiden: Brill, 2014), 191–194.
52. Judith Farr with Louise Carter, The Gardens of Emily Dickinson (Cambridge, MA: Harvard University Press, 2004), 3.
53. Jamaica Kincaid, My Garden (Book): (New York: Farrar, Strauss, Giroux, 1999), 153 , 166. Reading this book together with Kincaid’s likewise hybrid travel-garden-autobiographical volume Among Flowers (2005), Julietta Singh posits that the author/narrator’s overt self-contradictions is staging a productively fractured sense of postcolonial subjectivity, “a promise of stalling mastery” over plants and people alike in Unthinking Mastery: Dehumanism and Decolonial Entanglements (Durham, NC: Duke University Press, 2018), 161.
54. Reginald Campbell Thompson, The Assyrian Herbal: A Monograph on the Assyrian Vegetable Drugs (London: Luzac, 1924); and Rebecca Armstrong, Vergil’s Green Thoughts: Plants, Humans, and the Divine (Oxford: Oxford University Press, 2019), 2–3 .
55. Anne Van Arsdall, Medieval Remedies: The Old English Herbarium and Anglo-Saxon Medicine (New York: Routledge, 2002), xiii .
56. Gloria Anzaldúa, Borderlands/La Frontera: The New Mestiza (San Francisco: Aunt Lute Books, 1987).
57. Alice Walker, In Search of Our Mothers’ Gardens (New York: Harcourt, Brace, Jovanovich, 1983).
58. Randy Laist, “Introduction,” in Plants and Literature: Critical Plant Studies , ed. Randy Laist (Leiden, The Netherlands: Brill, 2013), 10.
59. Ryan, Plants in Contemporary Poetry , 15.
60. Monica Gagliano, John C. Ryan, and Patrícia Vieira, “Introduction,” The Language of Plants: Philosophy, Science, Literature , ed. Monica Gagliano, John C. Ryan, and Patrícia Vieira (Minneapolis: University of Minnesota Press, 2017), xi.
61. Joela Jacobs and Isabel Kranz, eds., Das Literarische Leben der Pflanzen: Poetiken des Botanischen , special issue, Literatur für Leser 40, no. 2 (2017): 85–90.
62. Mahood, Poet as Botanist .
63. Charlotte Otten, Environ’d with Eternity: God, Poems, and Plants in Sixteenth and Seventeenth Century England (Lawrence, KS: Coronado Press, 1984) .
64. Recently Ryan himself pursues the question of “what Latin American literature scholar Stephen F. White has described [as] ‘ethnobotanical poetry’ [or] . . . that which narrativises cultural knowledge of plants” in Ed. John Charles Ryan, “ Editorial: Plant Poetics ,” Plant Poetics , special issue, Plumwood Mountain: An Australian Journal of Ecopoetry and Ecopoetics 7, no. 1 (2020).
65. Miller, The Vegetative Soul , 183.
66. Sam George, Botany, Sexuality, and Women’s Writing, 1760–1830: From Modest Shoot to Forward Plant (Manchester, UK: Manchester University Press, 2007), 6 .
67. Isabel Kranz, “The Language of Flowers in Popular Culture and Botany,” in The Language of Plants: Philosophy, Science, Literature , ed. Monica Gagliano, John C. Ryan, and Patrícia Vieira (Minneapolis: University of Minnesota Press, 2017), 193; and Vanessa Diffenbaugh, The Language of Flowers (New York: Ballantine Books, 2011).
68. George, Botany, Sexuality , 107.
69. Isabel Kranz, “Slowly Unfolding: Molly Peacock Reads Mary Delaney’s Flowers,” Antennae: The Journal of Nature in Visual Culture 50 (2020): 22; and Molly Peacock, The Paper Garden: An Artist Begins Her Life’s Work at 72 (New York: Bloomsbury, 2010).
70. Novalis (Friedrich von Hardenberg), Heinrich von Ofterdingen (Berlin: Realschulbuchhandlung, 1802).
71. Theresa Kelley, Clandestine Marriage: Botany and Romantic Culture (Baltimore: Johns Hopkins University Press, 2012), 14 .
72. Alan Bewell, “Keats’s Realm of Flora,” Studies in Romanticism 31, no. 1 (1992): 71.
73. Natania Meeker and Antónia Szabari, Radical Botany: Plants and Speculative Fiction (New York: Fordham University Press, 2019), 2 .
74. Gilles Deleuze and Félix Guattari, A Thousand Plateaus , trans. Brian Massumi (Minneapolis: University of Minnesota Press, 1987), 11.
75. Meeker and Szabari, Radical Botany , 201.
76. Schiebinger, Plants and Empire , 4.
77. Aaron Pinnix, “Sargassum in the Black Atlantic: Entanglement and the Abyss in Bearden, Walcott, and Philip,” Atlantic Studies 16, no. 4 (2019): 423.
Related Articles
- American Nature Writing
- Actor–Network Theory
- Ecocriticism
Printed from Oxford Research Encyclopedias, Literature. Under the terms of the licence agreement, an individual user may print out a single article for personal use (for details see Privacy Policy and Legal Notice).
date: 23 April 2024
- Cookie Policy
- Privacy Policy
- Legal Notice
- Accessibility
- [66.249.64.20|45.133.227.243]
- 45.133.227.243
Character limit 500 /500
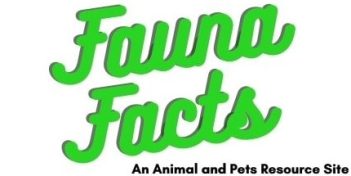
Plant and Animal Classification
(a beginner’s guide).

Plants and animals are classified using the Linnaean taxonomy. It is a hierarchy system that organizes living things into groups based upon common features.
The system operates on a 8-step cascading hierarchy that branches off from the top category (’domain’), which separates all living things into three buckets, down to ‘species’, which indicates a specific animal.
The eight levels (or ‘taxa’) of the hierarchy are shown below:
- Phylum/Division
This taxonomy was first proposed by Carl Lannaeus in his 1735 book Systema Naturea . Despite several modern adaptations, the Linnaean taxonomy remains the foundation for our current understanding of the biological relationships between living things.
Biological Taxonomic Classification Explained
Plural: Domains
The domain is the top taxa in the biological classification system and sits above the kingdom taxa.
There are three domains of life. They include Bacteria, Archaea, and Eukarya.
Bacteria and Archaea are buckets that hold two broad types of microorganisms (often single-celled) whose cells do not have membranes around the nucleus.
Eukarya is the bucket that holds all life with a complex cell that has a membrane, nucleus, and organelles. Animals and plants both fit into the Eukarya bucket.
Linnaeus didn’t actually come up with the ‘domain’ taxa. It was added to the Linnaean taxonomy by Carl Woese, Otto Kandler and Mark Wheelis in 1990.
Plural: Kingdoms
The kingdom is the second taxa in the biological classification system . It sits below domain and above phylum. There are six kingdoms.
Below the domain level, living organisms branch out into six kingdoms. These kingdoms reveal the basic different types of living things: animals, plants, fungi, protozoa (single-celled organisms), Chromista, and Bacteria.
Linnaeus only proposed two kingdoms (plants vs animals). This was replaced by Whittaker’s five kingdom model in 1968 and then Cavalier-Smith’s six kingdom model in 1998.
Here are the six kingdoms:
- The Animal Kingdom (Animalia) : This kingdom contains all multi-cellular organisms that (with some exceptions) can move around, breathe, eat, and reproduce sexually. There are over 1.5 million named animal species in the world today. This includes everything from humans to ants to jellyfish.
- The Plant Kingdom (Plantae) : The plant kingdom includes most multi-cellular organisms that generate energy through sunlight via a process called photosynthesis. They generally cannot move and more produce seeds as part of their reproduction. Examples include sunflowers, ferns, and pine trees.
- Fungi: Fungi used to be considered part of the plant kingdom but are now considered their own kingdom. They’re unique because they don’t get energy from the sun via photosynthesis, but actually digest other organic matter (they eat!). Examples include mushrooms, molds, and yeasts.
- Protista: Protista are a class of organisms that don’t fit well within any other category, so they sit into this ‘leftover’ category that was named by its founder the “primitive forms” of life. They’re single-celled organisms. An example is algae.
- Eubacteria – Eubacteria and archaebacteria (discussed next) are two separate types of bacteria. Eubacteria are single-celled organisms that live all around us. There are natural eubacteria in our food (especially yoghurt) and our intestines.
- Archaea – Archaea are the oldest living single-celled organisms and believed to be the original lifeforms. They were originally only believed to be found in extreme environments like hot springs, but scientists have now discovered them all over the place. We call the archaea who live in extreme environments “extremophiles” and study them to try to find out how life began.
3. Phylum / Division
Plural: Phyla / Divisions
Phylum and division are the third taxa in the biological classification system . They sits below kingdom and above class. We use ‘phylum’ for animals and ‘divison’ for plants. There are 7 animal phyla and 12 plant divisions.
3.1 Animals Example
There are 7 known animal phyla. Some recognizable ones are:
- Porifera – sponge-like animals found in the ocean (like spongebob squarepants!)
- Cnidaria – Marine animals like jellyfish, sea anemone, and coral. The world register of marine species [ 1 ] lists over 11,000 varieties.
- Mollusca – Soft invertebrates such as snails, octopi, oysters, and squid. There are over 85,000 known species [ 2 ] of mollusca.
- Arthropoda – These are invertebrate animals that hold themselves up with exoskeletons rather than bones. Examples include spiders, crabs, lobsters, centipedes, and scorpions.
- Chordata – These are vertebrates, meaning animals with skeletons. Most large animals fit into this category, including humans, dogs, alligators, and bears.
3.2 Plants Example
There are 12 known plant divisions. Some recognizable ones are:
- Bryophyta – includes mosses and liverworts
- Filicinophyta – includes ferns such as the lady fern, silver fern, and horsetail
- Coniferophyta – includes cone bearing trees such as the spruce, larch, pine, and fir trees
- Angiospermophyta – includes flowers such as the rose, sunflower, and lupin
Note: Botanists are increasingly tuning to ‘clades’ rather than domains to categorize plants. Domains focus on common features, while clades focus on common ancestry. Unfortunately, there is no clear taxonomy of clades at this time.
Plural: Classes
Class is the fourth taxa in the biological classification system . It sits below phylum and above order.
The ‘class’ taxa is where things get interesting as we can start to see distinct clusters of recognizable lifeforms at this level. We’ll focus specifically on chordata (vertebrate animals) and Angiospermophyta (flowering plants) here.
4.1 Animals Example
The phylum Chordata (commonly known as vertebrates) splits off into seven classes. Some of the most interesting classes within this phylum are:
- Amphibians – Cold-blooded vertebrates that live both in and out of water. Examples include frogs, toads, salamanders, newts.
- Reptiles – Cold-blooded vertebrates that (usually) live on land, including lizards, snakes, turtles, and crocodiles.
- Birds (aves) – Warm-blooded, feathered animals that lay hard eggs and (usually) are able to fly.
- Mammals – Warm-blooded animals that usually have fur or hair and produce milk to feed their young. This includes humans as well as cows and dogs.
4.2 Plants Example
The phylum Angiospermophyta (which are flowering plants) splits off into two classes: monocots and dicots.
- Monocots – Monocots start out with a singular-part seed, have a branching root system, and parallel veins on their leaves. But the easiest way to identify them is by looking at the petals on their flower. If the flower has petals in multiples of three (3 or 6 petals), they’re a monocot! Examples include irises and trilliums.
- Dicots – Dicots start out with a two-chambered seed, have a tap root system, and leaves that branch out like a maple leaf. Identify them by looking at their flowers which do not have petals in multiples of 3. Examples include roses, sunflowers, and violets.
As with domains, classes are increasingly less common in categorizing plants. These are being replaced with clades (groupings of plants with common ancestors). You may therefore see domains and classes either skipped entirely, or replaced with a list of ‘clades’ when categorizing plants.
Plural: Orders
Order is the fifth taxa in the biological classification system . It sits below class and above family.
We break down classes into orders. Let’s take a look again at one example from animals and one from plants.
5.1 Animals Example
The animals class known as mammals can be broken down into over 25 different orders. Here are some recognizable ones:
- Carnivora – Animals that mostly eat meat. This includes bears, dogs, tigers, hyenas, raccoons, and walruses.
- Rodentia– Rodents account for 40% of all mammals and are identifiable for the way they gnaw when they eat. Examples include squirrels, rats, mice, and beavers.
- Chiropptera – Better known as bats, these are the only mammals that can fly!
- Primates – Primates have advanced hands and feet (usually with opposable thumbs) and large brains, making them the most advanced of all animals. Humans, monkeys, gorillas, and orangutans are all primates.
5.2 Plants Example
The plants class known as dicots (one of the two classes of flowering plants) can be further broken down into dozens of different orders. Here are some recognizable ones:
- Rosales – You may recognize the root latin term for ‘rose’ in this name. This order includes roses, but also many other recognizable flowering plants, like strawberries, raspberries, pears, apricots, and elms.
- Geraniales – You may think of ‘geraniums’ when you read this order, and indeed geraniums are a genus of the geraniales order. Bridal wreaths are another common plant in this order. Many Geraniales plants are used to make perfumes.
- Sapindales – Genera and species of spindales include maple leaves, cashews, mangoes, and citrus fruits.
- Asterales – Common asterales you might find in your garden include daisies, sunflowers, and marigolds.
Plural: Families
Family is the sixth taxa in the biological classification system . It sits below order and above genus.
Below orders are families. Families are groups of animals that are usually observably related to the casual observer. Here are some examples from the animal and plant kingdoms.
6.1 Animals Example
These are examples of animal families within the carnivora order:
- Canidae – The canine family fits includes dogs, wolves, foxes, and coyotes.
- Felidae – The feline family includes cats, lynx, hyenas, tigers, lions, and jaguars.
- Ursidae – The Ursidae are bears and under this family exist multiple species including the grizzly bear, black bear, polar bear, and giant panda.
- Procyonidae – The Procyonidae family includes ringtails, raccoons, and olingos. There are many Procyonidae species in South America.
6.2 Plants Example
These are examples of plant families within the Rosales order:
- Urticaceae – This family within the Rosales order houses all 2,625 known species of nettles.
- Moraceae – The Moraceae family houses over 1,100 known species of plants including figs and mulberries.
- Rosaceae – The Rosaceae family, commonly known as the rose family, does house all known roses, but also houses many common fruits including apricots, apples, pears, cherries, and peaches.
Plural: Genera
Genus is the seventh taxa in the biological classification system . It sits below family and above species.
At the genus level, we’re getting specific enough that a species’ latin binomial name will contain the genus as the first word.
For example, the wolf’s scientific binomial name is Canis lupis . Here, we can instantly tell that it belongs to the genus Canis , while its species name is lupis . Always write the genus with a capital letter and italicize the full scientific name.
7.1 Animals Example
The following are genera from the family Felidae:
- Felis – The Felis genus houses closely related species including the domestic cat ( Felis catus ), European wildcat ( Felis silvestris ), African wildcat ( Felis lybica ), and Sand cat ( Felis margarita ).
- Leopardus – The Leopardus genus houses closely related species including the ocelot ( Leopardus pardalis ), Andean mountain cat ( Leopardus jacobita ), and Southern tiger cat ( Leopardus guttulus ).
- Lynx – The lynx genus houses closely related species including the Eurasian lynx ( Lynx lynx ), bobcat ( Lynx rufus ), Canadian lynx ( Lynx canadensis ), and Iberian lynx ( lynx pardinus ).
- Puma – The puma genus houses just one species: the cougar ( Puma concolor ).
- Panthera – Includes tigers ( Panthera tigris ), lions ( Panthera leo ), and jaguars ( Panthera onca ).
7.2 Plants Example
The following are genera from the family Rosaceae:
- Malus – Includes the trees of all species of apple, including the common orchard apple ( Malus domesticus ) and sweet crabapple ( Malus coronaria )
- Rosa – Includes all species of roses, including the common rose ( Rosa rosa ) and a white Chinese species ( Rosa laevigatae)
- Fragaria – This genus contains the plants of all 20 species of strawberries but no other berries.
- Prunus – This genus includes numerous edible fruits including plum trees (such as Prunus americana ), cherry tree (such as Prunus avium ), peach trees (such as Prunus persica ), and apricot trees (such as Prunus armeniaca ).
- Rubus – Includes many delicious berry plants including the raspberry plant (e.g. Rubus idaeus ), blackberry plant (e.g. Rubus laciniatus ), and dewberry plant (e.g. Rubus aboriginum ).
8. Species
Plural: Species
Species is the last taxa in the biological classification system . It sits below genus.
The species is the exact animal or plant. This is the lowest level on the biological taxonomy.
We can generally identify a species because all animals within the species share a fundamentally similar DNA sequence and are able to breed with one another.
However, there are some instances of interbreeding at the higher taxa of genus. Different species within the Canis genus, for example, can interbreed, which leads to hybrid animals such as Coywolves (a cross between a coyote and a wolf).
8.1 Animals Example
The following bears belong to the genus Ursus , which is a step under the family Ursidae (Bear).
- Polar Bear – The polar bear’s species is U. maritimus and its binomial name is Ursus maritimus .
- Black Bear – The American black bear’s species is U. maritimus and its binomial name is Ursus maritimus .
- Brown Bear – The brown bear’s species is U. arctos and its binomial name is Ursus arctos because it belongs to the Ursus genus. The North American grizzly bear ( Ursus arctos horribilis ) belongs to this species as they share genes and can interbreed.
Note that the giant panda ( Ailuropoda melanoleuca ), sun bear ( Helarctos malayanus ) and spectacled bear ( Tremarctos ornatus ) are not in the above list because they belong to the same bear family (Ursidae) but different genera ( Ailuropoda , Helarctos and Tremarctos respectively).
8.2 Plants Example
The following plants belong to the genus Prunus:
- Plum Trees – There are several species of plum tree. The common American plum tree is P. americana and its binomial name is Prunus americana .
- Cherry Trees – There are several species of cherry trees, such as the common P. avium , with the binomial name Prunus avium .
- Peach Trees – There are also several species of peach trees, such as the species P. persica , with the binomial name Prunus persica .
- Apricot Trees – The common American apricot tree is P. armeniaca , with the binomial name Prunus armeniaca .
Animal Classification Examples
1. american black bears, 3. grey wolves, 4. brown recluse spider, plant classification examples, 1. sunflowers, 2. scotch pine trees, 3. domestic apple, 4. western rose.
*As our understanding of plants evolves, classifications at the level of domain and class are becoming less valuable for describing plant groupings. It may be more useful to examine clades (groups of plants with common ancestors which do not fit neatly onto the Linnaean taxonomy) at the higher taxa for plants.
The Linnaean taxonomy enables scientists to categorize animals based on genetic similarities and relationships. This allows us to gain a better understanding of evolutionary theory, the movement and spread of animals over time, and relationships between current animals and extinct species. Knowledge of this taxonomy also helps laypeople develop a deeper understanding of animals and their relationships to one another when their binomial names are used in news and research reports.
Replacing Animal Testing with Stem Cell-Organoids : Advantages and Limitations
- Open access
- Published: 19 April 2024
Cite this article
You have full access to this open access article
- Guiyoung Park 1 ,
- Yeri Alice Rim 2 , 3 , 4 ,
- Yeowon Sohn 5 ,
- Yoojun Nam ORCID: orcid.org/0000-0003-4583-3455 5 , 6 &
- Ji Hyeon Ju 2 , 3 , 4 , 6
95 Accesses
Explore all metrics
Various groups including animal protection organizations, medical organizations, research centers, and even federal agencies such as the U.S. Food and Drug Administration, are working to minimize animal use in scientific experiments. This movement primarily stems from animal welfare and ethical concerns. However, recent advances in technology and new studies in medicine have contributed to an increase in animal experiments throughout the years. With the rapid increase in animal testing, concerns arise including ethical issues, high cost, complex procedures, and potential inaccuracies.
Alternative solutions have recently been investigated to address the problems of animal testing. Some of these technologies are related to stem cell technologies, such as organ-on-a-chip, organoids, and induced pluripotent stem cell models. The aim of the review is to focus on stem cell related methodologies, such as organoids, that can serve as an alternative to animal testing and discuss its advantages and limitations, alongside regulatory considerations.
Although stem cell related methodologies has shortcomings, it has potential to replace animal testing. Achieving this requires further research on stem cells, with potential societal and technological benefits.
Graphical Abstract
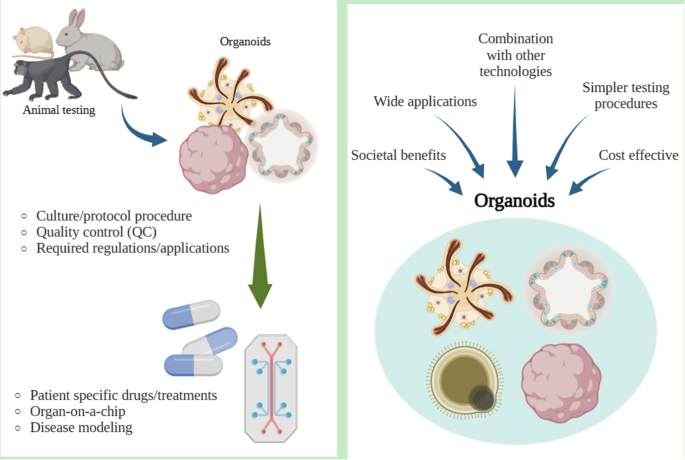
Avoid common mistakes on your manuscript.
Introduction
Historically, animal models have contributed substantially to the advancement and study of vaccines, surgical techniques, and various scientific experiments [ 1 ]. However, owing to the problems associated with animal testing, researchers are now questioning whether animal models and tests are the best options for these procedures. Growing animal testing is ethically concerning amid scientific evolution. According to the Humane Society International Organization, more than 100 million animals are killed annually worldwide for scientific purposes (Humane Society International). The animals used vary depending on their traits and include rats, mice, rabbits, dogs, cats, guinea pigs, zebrafish, swine [ 2 , 3 ].
In December 2022, the U.S. Food and Drug Administration (FDA) announced animal testing is no longer mandatory safety approval of products [ 4 ]. However, products that are used on the human body still require safety testing. In other words, testing for toxicity, compatibility, and safety is compulsory for products; however, animal testing is unnecessary for conducting these tests. In response, research facilities and companies have introduced alternatives such as computer simulations and in silico models. Stem cell therapy has gained popularity throughout the medical field, and various studies are underway to gain deeper knowledge [ 5 ]. With the emergence of this stem cell-based test, alternative methods have also arisen, potentially offering to become a replacement for animal testing.
When comparing test options, alternatives offer more beneficial attributes than animal testing. Non-animal tests are cost-effective, less time-consuming, and simpler procedures than animal tests [ 6 ]. However, most research institutions use animal models. This is because animal testing has been a longstanding experimental approach for decades [ 7 , 8 ]. Efforts are being made to replace animal testing with the use of human cells, as animal testing results often exhibit interspecies differences with humans, thus lacking the ability to reliably predict clinical outcomes. Application of advancing stem cell technology continue, but completely replacing animal experimentation poses significant challenges. Therefore, it is important to conduct further studies to advance the science of alternative testing methods. This review aimed to summarize the use of stem cell technology as an alternative to animal testing and discuss its advantages and limitations.
Current State of Animal Testing
Uses of animal testing.
Animal testing has been used for decades, and in the 21st century, the number of tests has increased considerably [ 2 ]. With approximately 100 million animals used for testing annually worldwide, science has been rapidly evolving. The primary function of animal testing is to test drugs, their toxicity, and their compatibility with the human body to ensure safe use. Hence, pre-launch testing is crucial. Companies and research facilities must subject their products to clinical trials before introducing them to potential customers.
Neurological disorder such as Parkinson’s and Alzheimer’s have also been modeled in animals to understand their mechanisms and to determine suitable treatments [ 9 , 10 , 11 ]. For instance, in the case of Parkinson’s disease, various animal models have been employed, including Caenorhabditis elegans, Zebrafish, and mice. Additionally, genetically modified mice carrying mutations associated with proteins like α-synuclein, Parkin, Pink1, and LRRK2, as well as mice induced with α-Synuclein Pre-Formed Fibril (PFF), are utilized to assess dopaminergic neuronal loss and investigate changes in α-synuclein aggregation. In Alzheimer’s disease, transgenic mice carrying mutations associated with familial Alzheimer’s disease (FAD), such as the 5xFAD model, are commonly used. These models allow for the evaluation of amyloid beta reduction through histological methods and the assessment of drug efficacy using behavioral tests like the Maze, providing insights into underlying disease mechanisms. Animals utilized as disease models contribute significantly to our comprehensive understanding of the mechanisms behind various illnesses, facilitating our grasp of these conditions. Research conducted using these animal disease models has indeed contributed to the discovery and development of treatments. However, it’s scientifically crucial to acknowledge that these animal models often present disparities in lifespans compared to humans and may not entirely mirror the intricate etiology of human diseases. Additionally, while animal experimentation is utilized for various conditions such as cancer, diabetes mellitus, and traumatic brain injury, it’s constrained by its inability to fully capture the nuances of the human immune system and intricate disease mechanisms (Table 1 ).
In addition to modeling diseases, animals are also used to test cosmetics or healing rates of products. In the cosmetics industry, animals are typically used to test skin or eye irritation to assess the safety of these products in humans [ 17 , 18 ]. The Draize test, developed in 1944 to test for such hazards in rabbits [ 19 ], is used to test products such as drugs and balms for wound healing. It involves creating wounds on animals to gauge recovery rates [ 16 ].
Related laws, Guidelines, and Principles
As of 2023, current regulations state that the FDA no longer deems animal tests necessary for evaluating product safety [ 4 ]. This enables companies and research facilities to explore possible non-animal testing when obtaining product approval. Additionally, out of 195 countries worldwide, only 42 have laws or regulations limiting animal testing for products (The Humane Society). Animal testing laws have been implemented by banning animal testing or limiting its use during testing. Europe completely banned cosmetics tested on animal testing in 2013 [ 3 , 20 , 21 ]. This demonstrates a push to limit animal testing; however, the movement remains ineffective because of the absence of laws against animal testing in most countries.
Guidelines for animal experimentation and clinical trials for drug development and safety testing have varied procedures among companies and researchers up to now. So, the Guidance for Industry for Preclinical Safety Evaluation of Biotechnology-Derived Pharmaceuticals from the Center for Drug Evaluation and Research provides guidelines for the safety assessment of products compiled from regulatory standards of several countries. According to these guidelines, preclinical trial researchers should consider factors such as animal species, age, delivery method (dosage, administration, treatment regimen, etc.), and test material stability [ 22 ] (Fig. 1 ).
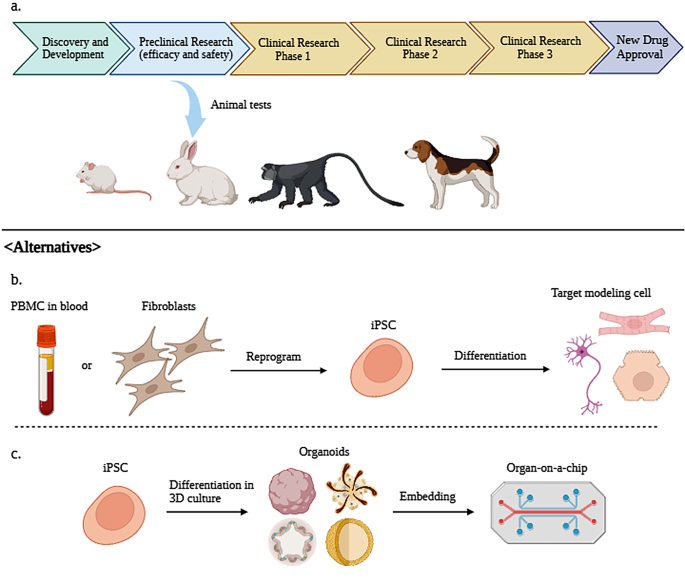
( A ) Procedure of new drug approval as stated by the Food and Drug Administration (FDA). In the preclinical research stage, small, medium, and large animals are usually used for testing new drugs. ( B ) iPSCs that can replacing animal testing. PBMCs or fibroblasts are reprogrammed to iPSCs and subsequently differentiated into target modeling cells such as neurons, cardiomyocytes, and hepatocytes. ( C ) iPSC-derived 3D organoids enable in vitro efficacy and safety testing. Organ-on-a-chip embedded with organoids used in in vitro tests, created using BioRender
The FDA has also provided a drug development process that includes these steps. The first step in drug development is discovering and researching a new drug (discovery and development stage). The second stage is preclinical research, in which drugs have to undergo a series of animal tests (or alternative tests, if possible) for safety. The FDA strongly suggests that animal preclinical trials follow Good Laboratory Practice (GLP). The main elements of GLP are as follows [ 23 ]: appropriate use of qualified personnel, quality assurance, appropriate use of facility and care for animals, proper operating procedures for animals used in trial, individual animal data collection and evaluation, testing product properly handled and analyzed, study proceeds with an approved protocol, data should be collected as outlined in the protocol, and full report prepared after procedures.
To enhance clinical translation, reproducibility issues in preclinical trials, such as biased allocation, insufficient controls, and lack of interdisciplinary, uncharacterized, or poorly characterized supplies [ 24 ]. The third step involves clinical testing on humans to assess safety and efficacy. The fourth and fifth stages comprise FDA post-market safety monitoring for all approved drugs [ 25 ].
Guidelines also suggest the 3R (replacement, reduction, and refinement) principle, which recommends that scientists follow certain criteria during clinical trials. Replacement involves using other testing methods other than animal testing [ 26 ]. In computer models, tissues, or stem cell research, if alternatives to animal testing exist, researchers should prioritize their use. Reduction involves minimizing the number of animal tests [ 26 ]. Questioning the necessity of animal tests during a particular part of our research and reducing their numbers imbues the concept with meaning. Refinement focuses on minimizing stress and providing the best care to animals [ 26 ], including providing proper food, entertainment, and clean well-maintained shelters.
As International efforts for animal replacement methods, research and development into alternative testing methods is already underway in both Europe and the United States, with each regulatory body establishing its own initiatives. In Europe, the European Center for the Validation of Alternative Methods (ECVAM) was founded in 1992, and since 2013, the sale of cosmetics containing ingredients tested on animals has been completely banned. Moreover, there are plans to expand the scope to include medical devices, health supplements, and pharmaceuticals in the future. In the United States, the Interagency Coordinating Committee on the Validation of Alternative Methods (ICCVAM) was established in 2000. The objective is to reduce animal testing by 2025 and eliminate mammalian animal testing entirely by 2035 through innovative advancements in alternative testing methodologies. In 2022, amendments to the Food, Drug, and Cosmetic Act in the United States removed mandatory animal testing requirements in the drug development stage and presented alternative testing methods as viable non-clinical trial options.
Problems/limitations of Animal Testing
A pressing issue with animal testing is the ethical concerns stemming from it. Most studies have demonstrated that these models undergo invasive procedures that often result in pain or even death. Research indicates that animals share pain and emotional capacity with humans [ 27 ]. Thus, sacrificing them for research can appear cruel. Advocates call for equitable treatment, opposing animal testing as inhumane and cruel. Such ethical issues has always followed animal testing and are ongoing [ 28 ].
Moreover, some studies have indicated that animal testing is not an accurate model for medicines or substances, highlighting the need for accurate and efficient testing alternatives that are similar humans. The complexity of human disease mechanisms raises doubts whether animal models can accurately replicate them.
Physiological differences between animals and humans mean a product safe for animals may not guarantee human safety [ 29 ]. Interspecies differences have led to poor results in correlating animal testing with human outcomes, consequently causing several clinical trial failures [ 30 ]. Between 2010 and 2017, clinical trials for drugs had a greater chance of failing phase І, owing to safety and efficacy [ 31 ]. In addition, even if a product passes phase І there is still a 90% rate of failure while undergoing the necessary procedures [ 32 , 33 ]. Prolonged use of animal testing can ultimately endanger humans, as some drugs and products approved through trials were later deemed harmful. Concerns such as high cost and long laborious procedures will be discussed below.
Benefits of Replacing Animal Testing
The main benefits of replacing animal tests with alternatives are as follows: cost-effective, time efficient, less complex testing procedures, and societal benefits.
Stem cell modeling is less expensive than animal testing. The Draize test mentioned before costs approximately $1,800, whereas non-animal testing methods cost considerably less [ 6 ]. Affordable procedures offer renewed chances for past costly research to emerge. A decrease in the cost of procedures would facilitate new drug development, making opportunities for new technologies easier.
Animal testing requires prior preparation that is often complex and time consuming. Several guidelines of various organizations worldwide follow certain principles and procedures. For animal testing, factors such as providing clean and well-maintained shelters, food, necessary supplies for survival, and entertainment are laborious [ 26 ]. Alternatives are time-efficient and less laborious, simpler protocols, and fewer supplies to maintain procedures.
Alternatives to Animal Testing Related to Stem Cells
Organoids are organ-like structures derived from self-organizing stem cells in 3D cell cultures. They exhibit organ-specific characteristics and originate from stem cells undergoing self-organization [ 34 , 35 ]. . They are beneficial over previous 2D cell culture, as they can show near-physiological cellular composition and actions [ 36 ]. Organoids are typically established from embryonic stem cells (ESCs), human pluripotent stem cells (PSCs), and adult stem cells [ 37 , 38 , 39 ]. The potential of organoids as alternatives stems from their correlation with patient reactions to products such as drugs, indicating that they are a promising for rare diseases where clinical trials are impractical [ 39 ]. Organoids have a wide range of applications and are suitable for studies of infectious diseases, hereditary diseases, and toxicity, and can provide personalized medicine for individual patients [ 38 ].
Recent studies have shown that PSC organoids can form complex brain organoids that are useful for modeling traumatic brain injury [ 15 ]. Organoids derived from PSCs are of various types, including stomach, lung, liver, kidney, cerebral, and thyroid, and can contribute to organ failure or dysfunction. Cancer organoids are cultured from thin tumor sections, which are efficient for studying cancer syndromes [ 34 ]. Organoid studies on Alzheimer’s disease highlight the possibility of using familial or sporadic Alzheimer’s disease induced pluripotent stem cells (iPSCs) to model brain activity [ 40 ]. Thyroid follicles derived from hESCs have the potential to be used as organoids to treat hypothyroidism [ 41 ] (Table 2 ). Technology development of 3D bioprinting organoids is underway, promising better productivity. Bioprinting for organoids includes inkjet-based bioprinting, laser-assisted bioprinting, extrusion-based bioprinting, and photo-curing bioprinting [ 42 ]. Ongoing studies are also exploring 3D printing technology using organoids, offering the possibility of creating organs for patient-tailored services and toxicology research.
However, organoids still possess limitations that render them unsuitable tools to replace animal testing. Organoids lack of vasculature structure affects growth and maturation, leading to differences in behavior compared to the original tissue [ 59 ]. This may result in only partial replication, leading to an incomplete disease model [ 38 ]. Moreover, the complexity and heterogeneity of certain organs, such as the brain or immune system, pose challenges for complete replication in organoid models. This inability to replicate such complexity can affect the translatability of findings from organoid studies to clinical applications. Research and experiments involving organoids often require lengthy culture protocols, which can vary depending on the type of organoid being cultivated. In some extreme cases, organoid culture may extend for months or even years, as seen in examples such as intestinal organoids(8 weeks or more), retinal organoids(6 ~ 39 weeks or more), brain organoids(12 weeks or more), and liver organoids(4 ~ 8 weeks or more) [ 60 , 61 , 62 , 63 , 64 ]. Even after going through the lengthy process, there are sometimes a lack of established organoids in sufficient numbers. This limited availability of organoids can hinder the procedure of functional testing, which can lead to insufficient research outcomes. Organoids also lack the intricate network of connections that can be seen in living organisms. Inter-organ communication is crucial when checking metabolic health, and with organoids lacking such an important factor, it is difficult to create treatments for any abnormalities regarding infection and diseases. Organoids also lack a diverse set of cell types, structural organization, and physiological functions in comparison to functioning organs, which limits the ability to accurately replicate disease processes and responses to treatment [ 59 ]. When compared to animal models, organoids fall behind, as animal models offer a broader view of processes for diseases, immune responses, and systemic effects of treatments. Another noteworthy concern arises from the fact that current production technology for organoids under GMP (Good Manufacturing Practice) standards has yet to be established.
Quality Control of Organoid
For organoids to serve as suitable models for diseases or experimental purposes, quality control (QC) is essential. Accuracy and consistency in production lead to more precise results, ensuring better therapeutic treatments or modeling. If quality control for organoids isn’t established sufficiently, problems such as inconsistent test results, misinterpretation of existing data, wastage of valuable resources, reproducibility issues, unreliable models, and ethical concerns regarding biomedical studies could arise.
Organoid structures and functions can be assessed through multiple methods. Structural assessment of organoids can be performed using bright-field imaging for both quantitative and qualitative research. Additionally, methods such as immunofluorescent staining, transmission electron microscopy, and scanning electron microscopy are also utilized [ 65 , 66 ]. The functionality of organoids can be assessed through qPCR and single-cell or bulk cell RNA sequencing, which provide quantitation of marker gene expression, revealing cell identity and composition [ 67 ]. Assay methods like ELISA and colorimetric assays are useful for secretome quantification while Luciferase essays help measure enzyme activity [ 65 , 68 ]. Staining methods such as Glycosaminoglycan (GAG) staining(specifically for synovial mesenchymal stromal cell (SMSC) organoids), immunofluorescence staining, and Alizarin red staining mainly help with visualizing components within the organoid [ 65 , 68 , 69 ]. There are also more direct methods like implantation to test the in vivo functions of organoids [ 65 , 70 ] (Table 3 ).
Extracellular microenvironment, which contain such things as soluble bioactive molecules, extracellular matrix, and biofluid flow, contributes to the growth rate and formation of organoids. Given the variation in extracellular microenvironments across different types of organoids, it is imperative to modulate the extracellular microenvironment accordingly for each organoid type. This ensures the production of organoids with consistent quality across different production batches [ 71 ].
Regulations/Applications Regarding Organoids from the FDA
While there aren’t any specific regulations regarding organoids from the FDA(Food and Drug Administrations) as of in the recent years, there are two categories of applications that include framework for cell related therapies, which include organoids. There are two applications, Biologics License Application (BLA) and the Investigational New Drug (IND) Application. The BLA, as stated in the official website of FDA, is a request for permission to introduce and deliver for a biologic product(vaccines, somatic cells, gene therapy, tissues, recombinant therapeutic proteins, organoids, etc.) into interstate commerce. Requirements for a BLA includes applicant information, product/manufacturing information, pre-clinical studies, clinical studies, and labeling. The IND application is a request for authorization to administer an investigation drug or biological product to humans. IND had three types: Investigator IND, Emergency Use IND, and Treatment IND which could fall into two categories being commercial or non-commercial. The IND application must contain the following broad areas of information: Animal Pharmacology and Toxicology studies, Manufacturing Information, Clinical protocols and Investigator Information.
When examining the current ongoing clinical trials( ClinicalTrials.gov ) in the application of organoids, it can be noted that they are being utilized in refractory cancers, osteosarcoma, high-grade glioma, advanced breast cancer, and colorectal cancer. This pertains to the utilization of the organoid platform to investigate the sensitivity to various drugs (chemotherapy, hormonal therapy, targeted therapy) by exposing them to each individual agent (or combination of agents). It is anticipated and ongoing to aid in clinical decisions regarding the optimal treatment option for each patient.
Organ-on-a-chip
Organoid chips(OoC) can be regarded as the outcome of merging biology and microtechnology, serving as microfluidic cell culture devices [ 72 , 73 ]. OoC has the ability to mimic the cellular environment, which leads to an examination of their effects on cell communication with more accessibility and ease. The chips are generally designed by collecting cells (primary cells, transformed cell lines, human ESC, or iPSCs) using equipment with pumps(that enable fluid flow), incubators, sensors, and microscopes to monitor and examine the cells in the system [ 49 , 74 ] (Fig. 1 ). Depending on the type or cell or method cells can be aggregated in matrix or matrixless conditions [ 75 ].
Various types of human organ chips, including the liver, heart, eyes, kidneys, bones, intestines, and skin, are used to simulate the breathing motion. Single-organ chips such as liver-on-a-chip and lung-on-a-chip are useful for observing individual chemical reactions [ 53 ]. There are also multiple organ-on-chip, which are organ-chips connected to a vast system [ 76 ]. The main purpose of multi-organ-on-chips is to simulate the entire body, recognizing that a single organ does not represent the entire human system. Using multiple organ-on-chips connected to one system allows the analysis of how various organs communicate with each other.
The U.S. Food and Drug Administration (FDA) and the U.S. National Institutes of Health (NIH) have provided project support for tissue chips for drug screening, including lung-on-a-chip. Additionally, efforts are being made globally to advance the utilization of organoid chips, such as the establishment of the European Organ-on-Chip Society in Europe.
A limitation of OoCs is their complex experimental setup [ 77 ], which can be avoided with clear guidelines or protocols. Cell medium changes also raise concerns about chip environments [ 77 ]. There is also the issue of using animal models to validate OoC systems initially [ 78 ]. To address this, OoC experts recommend forming well-established collaborations with developers, toxicologists, and pharmaceutical companies to explore alternative solutions.
iPSCs(Induced Pluripotent stem Cells)
iPSCs are a recent development in the field of disease modeling. Having traits such as self-renewal and pluripotency, iPSCs can transform into various cells within the human body (Fig. 1 ); thus, reprogramming patient cells creates personalized medicine for specific diseases [ 79 , 80 ]. The ability to produce a large batch of iPSCs with only a small number of patient samples is important [ 81 , 82 ]. The objectives of iPSC models closely align with the 3R principle [ 83 ]. Replacing animal models in research while adhering to reduction and refinement principles is expected to be advantageous.
iPSCs are research to find cures for various diseases and are used as broad disease models (Table 2 ). For example, iPSCs from patients with Parkinson’s disease differentiate into midbrain dopaminergic neurons (DAns) in the substantia nigra pars compacta (SNpc), which can be used to model Parkinson’s disease on a cellular basis [ 43 , 44 , 45 ]. For cardiac diseases, which include a decrease in cardiomyocytes that leads to scar formation and ultimately heart function failure, there are existing studies that explore iPSCs for novel therapeutic cures [ 84 ]. iPSC-derived progenitors such as human HCN4 + and human ESC derived ROR2+, CD13+, KDR+, PDGFRα + cells later generate cardiomyocytes [ 47 ]. For cancer modeling using iPSCs, reprogrammed tumor specimens or iPSCs with premalignant or early genetic lesions can show the stages of cancer [ 49 ]. iPSCs from patients that are healthy and those with Alzheimer’s disease differentiate into the main brain cells, modeling the human brain with a functional blood barrier. Further research could drive drug discovery [ 9 ]. Studies of organ failure or dysfunction have shown that human iPSCs are useful. Research on lung regeneration has shown that endogenous and exogenous stem cells mediate therapeutic results [ 50 ]. Another study focused on the use of liver hepatoblasts, which could help alleviate hepatotoxicity through liver development and hepatic differentiation [ 85 ].
However, iPSCs are still in a relatively early developmental phase and have several limitations. Concerns for researchers regarding iPSCs is in vitro culture adaptation and tumorigenicity, the inability to completely reflect in vivo 3D environments, and the variation of differentiated cells depending on the protocol [ 86 , 87 ]. Quality control of differentiated cells and influencing factors are crucial for iPSC researchers, impacting their applicability as medical models or treatments.
Figure 2 Human diagram showing multiple stem cell-related technologies that can be applied to various human organs.
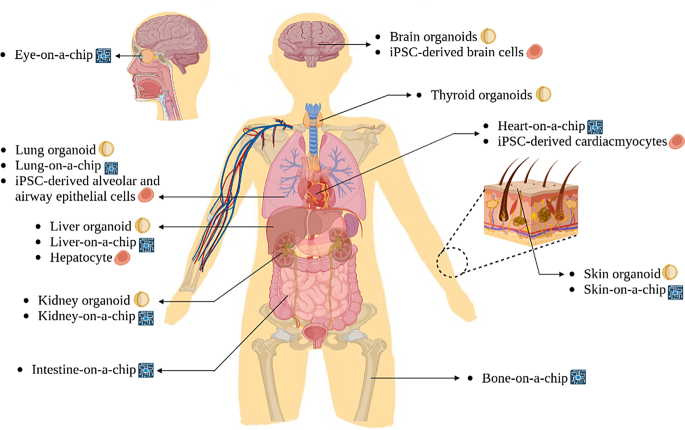
A BioRender diagram depicts diverse stem cell technologies for human organs
Limitations
Stem cell-related methodologies, such as organoids, are a very new technology in the field of animal alternative testing. In the early developmental stage, alternative stem cell models and technologies still require a few years of testing. Animal testing is still used today, owing to its historical role in safety and efficacy assessment. New alternatives have been presented; however, the uncertainty of these methods have caused most researchers to adhere to old protocols. In cases of complex diseases arising from various factors such as cardiovascular, neurodegenerative, and infertility, complete replacement by animal alternative testing methods may still be impractical. In such instances, it is crucial to concurrently employ animal experimentation alongside alternative testing methods utilizing organoids or stem cells to bolster data reliability. As a component of these endeavors, numerous researchers have undertaken disease modeling, such as stroke, utilizing brain organoids and cardiac organoids in in vitro experiments. The solution involves focusing on alternative testing methods [ 88 ]. By transforming old methods and creating alternatives, this shift could be the norm. There has already been a move toward that goal, as the FDA has established a cross-agency working group (The Alternative Methods Working Group) to promote various alternative methods, such as in vivo, in vitro, in silico , or system toxicology modeling [ 89 ]. In the 2021, FDA report titled “Advancing Regulatory Science at FDA,” the most prioritized area is identified as “Advancing Novel Technologies to Improve Predictivity of Non-clinical Studies and Replace, Reduce, and Refine Reliance on Animal Testing.”
Given ongoing research in alternative stem cell-related methods, this appears promising to replace animal testing. These alternatives offer advantages for scientists and the public. However, it is important to acknowledge that iPSCs, organoids, and OoCs each have distinct strengths and limitations. With continued advancements and studies to further understand these issues, these limitations can be avoided.
Data Availability
All data pertaining to this manuscript are included within the article.
Abbreviations
Food and Drug Administration
organ-on-chip
induced pluripotent stem cell
pluripotent stem cell
Embryonic stem cell
Center for Drug Evaluation and Research, GLP, Good Laboratory Practice
Dopaminergic neurons
Substantia Nigra pars compacta
Robinson, N. B., et al. (2019). The current state of animal models in research: A review. International Journal of Surgery , 72 , 9–13.
Article PubMed Google Scholar
Ericsson, A. C., Crim, M. J., & Franklin, C. L. (2013). A brief history of animal modeling. Missouri Medicine , 110 (3), 201–205.
PubMed PubMed Central Google Scholar
Balls, M. (2022). Alternatives to Laboratory animals: Trends in replacement and the three rs. Alternatives to Laboratory Animals , 50 (1), 10–26.
Han, J. J. (2023). FDA modernization Act 2.0 allows for alternatives to animal testing. Artificial Organs , 47 (3), 449–450.
Deinsberger, J., Reisinger, D., & Weber, B. (2020). Global trends in clinical trials involving pluripotent stem cells: A systematic multi-database analysis. Npj Regenerative Medicine , 5 (1), 15.
Article PubMed PubMed Central Google Scholar
Meigs, L., et al. (2018). Animal testing and its alternatives - the most important omics is economics. Altex , 35 (3), 275–305.
Horejs, C. (2021). Organ chips, organoids and the animal testing conundrum. Nat Rev Mater , 6 (5), 372–373.
Veening-Griffioen, D. H., et al. (2021). Tradition, not science, is the basis of animal model selection in translational and applied research. ALTEX , 38 (1), 49–62.
PubMed Google Scholar
Penney, J., Ralvenius, W. T., & Tsai, L. H. (2020). Modeling Alzheimer’s disease with iPSC-derived brain cells. Molecular Psychiatry , 25 (1), 148–167.
Khan, E., Hasan, I., & Haque, M. E. (2023). Parkinson’s Disease: Exploring Different Animal Model Systems . International Journal of Molecular Sciences , 24(10).
Chia, S. J., Tan, E. K., & Chao, Y. X. (2020). Historical Perspective: Models of Parkinson’s Disease . International Journal of Molecular Sciences , 21(7).
Li, Z., et al. (2021). Application of animal models in Cancer Research: Recent progress and future prospects. Cancer Manag Res , 13 , 2455–2475.
Article CAS PubMed PubMed Central Google Scholar
Kottaisamy, C. P. D., et al. (2021). Experimental animal models for diabetes and its related complications—a review. Laboratory Animal Research , 37 (1), 23.
King, A. J. (2012). The use of animal models in diabetes research. British Journal of Pharmacology , 166 (3), 877–894.
Ramirez, S. (2021). Modeling traumatic Brain Injury in Human Cerebral organoids . Cells , 10(10).
Grada, A., Mervis, J., & Falanga, V. (2018). Research Techniques made simple: Animal models of Wound Healing. The Journal of Investigative Dermatology , 138 (10), 2095–2105e1.
Article CAS PubMed Google Scholar
McNamee, P., et al. (2009). A tiered approach to the use of alternatives to animal testing for the safety assessment of cosmetics: Eye irritation. Regulatory Toxicology and Pharmacology , 54 (2), 197–209.
Macfarlane, M., et al. (2009). A tiered approach to the use of alternatives to animal testing for the safety assessment of cosmetics: Skin irritation. Regulatory Toxicology and Pharmacology , 54 (2), 188–196.
York, M., & Steiling, W. (1998). A critical review of the assessment of eye irritation potential using the Draize rabbit eye test. Journal of Applied Toxicology , 18 (4), 233–240.
Fentem, J. H. (2023). The 19th FRAME Annual lecture, November 2022: Safer Chemicals and Sustainable Innovation Will be achieved by Regulatory Use of Modern Safety Science, not by more animal testing. Alternatives to Laboratory Animals , 51 (2), 90–101.
Daneshian, M., et al. (2015). Animal use for science in Europe. Altex , 32 (4), 261–274.
Center for Drug Evaluation and, R., R. Center for Biologics Evaluation and, and H. International Conference on, Guidance for industry: S6 preclinical safety evaluation of biotechnology-derived pharmaceuticals (1997). Rockville, MD: U.S. Dept. of Health and Human Services, Food and Drug Administration, Center for Drug Evaluation and Research: Center for Biologics Evaluation and Research.
Centanni, J. M. (2017). Chap. 2 - Preclinical Animal Testing requirements and considerations . Mesenchymal stromal cells (pp. 37–60). Academic. S. Viswanathan and P. Hematti, Editors.
Ioannidis, J. P. A., Kim, B. Y. S., & Trounson, A. (2018). How to design preclinical studies in nanomedicine and cell therapy to maximize the prospects of clinical translation. Nat Biomed Eng , 2 (11), 797–809.
Van Norman, G. A. (2016). Drugs, devices, and the FDA: Part 1: An overview of approval processes for drugs. JACC Basic Transl Sci , 1 (3), 170–179.
Liebsch, M., et al. (2011). Alternatives to animal testing: Current status and future perspectives. Archives of Toxicology , 85 (8), 841–858.
Kiani, A. K., et al. (2022). Ethical considerations regarding animal experimentation. Journal of Preventive Medicine and Hygiene , 63 (2 Suppl 3), E255–E266.
Doke, S. K., & Dhawale, S. C. (2015). Alternatives to animal testing: A review. Saudi Pharm J , 23 (3), 223–229.
Akhtar, A. (2015). The flaws and human harms of animal experimentation. Cambridge Quarterly of Healthcare Ethics , 24 (4), 407–419.
Van Norman, G. A. (2020). Limitations of Animal studies for Predicting toxicity in clinical trials: Part 2: Potential Alternatives to the use of animals in preclinical trials. JACC Basic Transl Sci , 5 (4), 387–397.
Dowden, H., & Munro, J. (2019). Trends in clinical success rates and therapeutic focus. Nature Reviews. Drug Discovery , 18 (7), 495–496.
Takebe, T., Imai, R., & Ono, S. (2018). The current status of Drug Discovery and Development as originated in United States Academia: The influence of Industrial and academic collaboration on Drug Discovery and Development. Clinical and Translational Science , 11 (6), 597–606.
Sun, D., et al. (2022). Why 90% of clinical drug development fails and how to improve it? Acta Pharm Sin B , 12 (7), 3049–3062.
Dutta, D., Heo, I., & Clevers, H. (2017). Disease modeling in stem cell-derived 3D Organoid systems. Trends in Molecular Medicine , 23 (5), 393–410.
Wang, X. (2019). Stem cells in tissues, organoids, and cancers. Cellular and Molecular Life Sciences , 76 (20), 4043–4070.
Li, M., & Izpisua Belmonte, J. C. (2019). Organoids - Preclinical models of Human Disease. New England Journal of Medicine , 380 (6), 569–579.
Rookmaaker, M. B., et al. (2015). Development and application of human adult stem or progenitor cell organoids. Nature Reviews Nephrology , 11 (9), 546–554.
Clevers, H. (2016). Modeling Development and Disease with Organoids. Cell , 165 (7), 1586–1597.
Schutgens, F., & Clevers, H. (2020). Human organoids: Tools for understanding Biology and Treating diseases. Annual Review of Pathology: Mechanisms of Disease , 15 , 211–234.
Article CAS Google Scholar
Barak, M., et al. (2022). Human iPSC-Derived neural models for studying Alzheimer’s Disease: From neural stem cells to cerebral organoids. Stem Cell Reviews and Reports , 18 (2), 792–820.
Romitti, M., et al. (2022). Transplantable human thyroid organoids generated from embryonic stem cells to rescue hypothyroidism. Nature Communications , 13 (1), 7057.
Ren, Y., et al. (2021). Developments and opportunities for 3D Bioprinted Organoids. Int J Bioprint , 7 (3), 364.
Laperle, A. H., et al. (2020). iPSC modeling of young-onset Parkinson’s disease reveals a molecular signature of disease and novel therapeutic candidates. Nature Medicine , 26 (2), 289–299.
Avazzadeh, S. (2021). Modelling Parkinson’s Disease: iPSCs towards Better Understanding of Human Pathology . Brain Sci , 11(3).
Stoddard-Bennett, T., & Reijo Pera, R. (2019). Treatment of Parkinson’s Disease through Personalized Medicine and Induced Pluripotent Stem Cells . Cells , 8(1).
Funakoshi, S., & Yoshida, Y. (2021). Recent progress of iPSC technology in cardiac diseases. Archives of Toxicology , 95 (12), 3633–3650.
Matsa, E., Burridge, P. W., & Wu, J. C. (2014). Human stem cells for modeling heart disease and for drug discovery. Science Translational Medicine , 6 (239), 239ps6.
Hnatiuk, A. P., et al. (2021). Human iPSC modeling of heart disease for drug development. Cell Chem Biol , 28 (3), 271–282.
Papapetrou, E. P. (2016). Patient-derived induced pluripotent stem cells in cancer research and precision oncology. Nature Medicine , 22 (12), 1392–1401.
Aboul-Soud, M. A. M., Alzahrani, A. J., & Mahmoud, A. (2021). Induced Pluripotent Stem cells (iPSCs)-Roles in Regenerative therapies, Disease Modelling and Drug Screening . Cells , 10(9).
Sun, W., et al. (2019). Organ-on-a-Chip for Cancer and Immune organs modeling. Adv Healthc Mater , 8 (15), e1900754.
Wang, Y., et al. (2020). Dantrolene ameliorates impaired neurogenesis and synaptogenesis in Induced pluripotent stem cell lines derived from patients with Alzheimer’s Disease. Anesthesiology , 132 (5), 1062–1079.
Beckwitt, C. H., et al. (2018). Liver ‘organ on a chip’. Experimental Cell Research , 363 (1), 15–25.
Shi, W., et al. (2021). Design and evaluation of an in vitro mild traumatic brain Injury modeling System using 3D printed Mini Impact device on the 3D cultured human iPSC derived neural progenitor cells. Adv Healthc Mater , 10 (12), e2100180.
Lee, J., et al. (2020). Hair-bearing human skin generated entirely from pluripotent stem cells. Nature , 582 (7812), 399–404.
Jung, S. Y., et al. (2022). Wnt-activating human skin organoid model of atopic dermatitis induced by Staphylococcus aureus and its protective effects by Cutibacterium acnes. iScience , 25 (10), 105150.
Risueño, I., et al. (2021). Skin-on-a-chip models: General overview and future perspectives. APL Bioeng , 5 (3), 030901.
Aghmiuni, A. I., & Keshel, S. H. (2023). Chap. 1 0 - Eye-on-a-chip , in Principles of Human Organs-on-Chips , M. Mozafari, Editor. Woodhead Publishing. pp. 315–369.
Andrews, M. G., & Kriegstein, A. R. (2022). Challenges of Organoid Research. Annual Review of Neuroscience , 45 , 23–39.
Pleguezuelos-Manzano, C., et al. (2020). Establishment and culture of human intestinal organoids derived from adult stem cells. Current Protocols In Immunology / Edited By John E. Coligan. [Et Al.] , 130 (1), e106.
Wahle, P., et al. (2023). Multimodal spatiotemporal phenotyping of human retinal organoid development. Nature Biotechnology , 41 (12), 1765–1775.
Kathuria, A., et al. (2020). Comparative transcriptomic analysis of cerebral organoids and cortical neuron cultures derived from Human Induced Pluripotent Stem cells. Stem Cells and Development , 29 (21), 1370–1381.
Zhao, J., et al. (2020). APOE4 exacerbates synapse loss and neurodegeneration in Alzheimer’s disease patient iPSC-derived cerebral organoids. Nature Communications , 11 (1), 5540.
Zhu, X., et al. (2021). Liver organoids: Formation strategies and Biomedical Applications. Tissue Eng Regen Med , 18 (4), 573–585.
Zhao, Z. (2022). Organoids Nat Rev Methods Primers , 2.
Drakhlis, L., et al. (2021). Human heart-forming organoids recapitulate early heart and foregut development. Nature Biotechnology , 39 (6), 737–746.
Broutier, L., et al. (2016). Culture and establishment of self-renewing human and mouse adult liver and pancreas 3D organoids and their genetic manipulation. Nature Protocols , 11 (9), 1724–1743.
Sun, Y., et al. (2021). Generating 3D-cultured organoids for pre-clinical modeling and treatment of degenerative joint disease, in Signal Transduct Target Ther (p. 380). England.
Hemeryck, L., et al. (2022). Organoids from human tooth showing epithelial stemness phenotype and differentiation potential. Cellular and Molecular Life Sciences , 79 (3), 153.
Huch, M., et al. (2013). In vitro expansion of single Lgr5 + liver stem cells induced by wnt-driven regeneration. Nature , 494 (7436), 247–250.
Sullivan, K. M., et al. (2022). Extracellular Microenvironmental Control for Organoid Assembly. Tissue Eng Part B Rev , 28 (6), 1209–1222.
Low, L. A., et al. (2021). Organs-on-chips: Into the next decade. Nature Reviews. Drug Discovery , 20 (5), 345–361.
Bhatia, S. N., & Ingber, D. E. (2014). Microfluidic organs-on-chips. Nature Biotechnology , 32 (8), 760–772.
Leung, C. M., et al. (2022). A guide to the organ-on-a-chip. Nature Reviews Methods Primers , 2 (1), 33.
Olgasi, C., Cucci, A., & Follenzi, A. (2020). iPSC-Derived liver organoids: A journey from Drug Screening, to Disease modeling, arriving to Regenerative Medicine . International Journal of Molecular Sciences , 21(17).
Picollet-D’hahan, N., et al. (2021). Multiorgan-on-a-Chip: A systemic Approach to Model and Decipher Inter-organ Communication. Trends Biotechnol , 39 (8), 788–810.
Wang, H., et al. (2021). 3D cell culture models: Drug pharmacokinetics, safety assessment, and regulatory consideration. Clinical and Translational Science , 14 (5), 1659–1680.
Kang, S., Park, S. E., & Huh, D. D. (2021). Organ-on-a-chip technology for nanoparticle research. Nano Converg , 8 (1), 20.
Kim, C. (2015). iPSC technology–powerful hand for disease modeling and therapeutic screen. Bmb Reports , 48 (5), 256–265.
Qian, L., & Tcw, J. (2021). Human iPSC-Based modeling of central nerve System disorders for Drug Discovery . International Journal of Molecular Sciences , 22(3).
Gómez-Lechón, M. J., & Tolosa, L. (2016). Human hepatocytes derived from pluripotent stem cells: A promising cell model for drug hepatotoxicity screening. Archives of Toxicology , 90 (9), 2049–2061.
Blaszkiewicz, J., & Duncan, S. A. (2022). Advancements in Disease modeling and Drug Discovery using iPSC-Derived hepatocyte-like cells . Genes (Basel) , 13(4).
O’Connor, M. D. (2013). The 3R principle: Advancing clinical application of human pluripotent stem cells. Stem Cell Research & Therapy , 4 (2), 21.
Article Google Scholar
Parrotta, E. I. (2020). Modeling Cardiac Disease mechanisms using Induced Pluripotent Stem Cell-Derived cardiomyocytes: Progress, promises and challenges . International Journal of Molecular Sciences , 21(12).
Takebe, T., et al. (2013). Vascularized and functional human liver from an iPSC-derived organ bud transplant. Nature , 499 (7459), 481–484.
Lee, A. S., et al. (2013). Tumorigenicity as a clinical hurdle for pluripotent stem cell therapies. Nature Medicine , 19 (8), 998–1004.
Kramer, N., et al. (2016). Full biological characterization of human pluripotent stem cells will open the door to translational research. Archives of Toxicology , 90 (9), 2173–2186.
Balls, M., Bailey, J., & Combes, R. D. (2019). How viable are alternatives to animal testing in determining the toxicities of therapeutic drugs? Expert Opinion on Drug Metabolism & Toxicology , 15 (12), 985–987.
Administration, U. S. F. D. (2021). Advancing New Alternative Methodologies at FDA . Jan U.S. Food & Drug Administration: FDA website. pp. 1–34.
Download references
Acknowledgements
Not applicable.
This research was supported by a grant of the Korea Health Technology R&D Project through the Korea Health Industry Development Institute (KHIDI), funded by the Ministry of Health & Welfare, Republic of Korea (grant number : HI22C1314).
Author information
Authors and affiliations.
School of Biopharmaceutical and Medical Sciences, Health & Wellness College, Sungshin Women’s University, 55, Dobong-ro 76ga-gil, Gangbuk-gu, Seoul, Republic of Korea
Guiyoung Park
CiSTEM laboratory, Convergent Research Consortium for Immunologic Disease, Seoul St. Mary’s Hospital, College of Medicine, The Catholic University of Korea, Seoul, 06591, Republic of Korea
Yeri Alice Rim & Ji Hyeon Ju
Division of Rheumatology, Department of Internal Medicine, Seoul St. Mary’s Hospital, Institute of Medical Science, College of Medicine, The Catholic University of Korea, 4 3, Seoul, 06591, Republic of Korea
Department of Biomedicine & Health Sciences, Seoul St. Mary’s Hospital, College of Medicine, The Catholic University of Korea, Seoul, 06591, Republic of Korea
Department of Biohealth Regulatory Science, Sungkyunkwan University, Suwon, South Korea
Yeowon Sohn & Yoojun Nam
Yipscell Inc, L2 Omnibus Park, Banpo-dearo 222, Seocho-gu, Seoul, Korea
Yoojun Nam & Ji Hyeon Ju
You can also search for this author in PubMed Google Scholar
Contributions
GP designed the study and wrote the manuscript. YAR, YS and YN edited the manuscript. YN and JHJ approved the final manuscript. All the authors have read and approved the final draft of this manuscript.
Corresponding authors
Correspondence to Yoojun Nam or Ji Hyeon Ju .
Ethics declarations
Ethics approval and consent to participate, consent for publication, competing interests.
The authors declare that they have no competing interests.
Additional information
Publisher’s note.
Springer Nature remains neutral with regard to jurisdictional claims in published maps and institutional affiliations.
Rights and permissions
Open Access This article is licensed under a Creative Commons Attribution 4.0 International License, which permits use, sharing, adaptation, distribution and reproduction in any medium or format, as long as you give appropriate credit to the original author(s) and the source, provide a link to the Creative Commons licence, and indicate if changes were made. The images or other third party material in this article are included in the article’s Creative Commons licence, unless indicated otherwise in a credit line to the material. If material is not included in the article’s Creative Commons licence and your intended use is not permitted by statutory regulation or exceeds the permitted use, you will need to obtain permission directly from the copyright holder. To view a copy of this licence, visit http://creativecommons.org/licenses/by/4.0/ .
Reprints and permissions
About this article
Park, G., Rim, Y.A., Sohn, Y. et al. Replacing Animal Testing with Stem Cell-Organoids : Advantages and Limitations. Stem Cell Rev and Rep (2024). https://doi.org/10.1007/s12015-024-10723-5
Download citation
Accepted : 08 April 2024
Published : 19 April 2024
DOI : https://doi.org/10.1007/s12015-024-10723-5
Share this article
Anyone you share the following link with will be able to read this content:
Sorry, a shareable link is not currently available for this article.
Provided by the Springer Nature SharedIt content-sharing initiative
- Animal Testing Alternatives
- Animal Testing law
- Organ-on-chips
- Find a journal
- Publish with us
- Track your research
A Habitat for Humanity—And Plants and Animals, Too
Fab Tree Hab/Credit: Tracey Friedman
Goodbye, brick and mortar. Hello, wood and jute!
As we try to imagine the future of sustainable architecture, an innovative structure in New Windsor, NY, composed of approximately 70 living willow trees offers a compelling example of what that could look like. Standing at 32 feet, Fab Tree Hab is an expansive open-air canopy with trees growing upward along cross-laminated timber scaffolding. The trees are bound by a natural fiber called jute to increase their strength as they grow. Throughout Fab Tree Hab are “pockets” that serve as micro-habitats in this larger ecosystem that serves as a terrestrial reef—a land structure similar to a coral reef that enables flora and fauna to pass through or establish homes.
This project is the creation of the nonprofit organization Terreform ONE , a team of architects, designers, and artists led by Gallatin Associate Professor Mitchell Joachim . Joachim began considering such a structure about 20 years ago following a prompt from Habitat for Humanity to submit innovative designs for suburban housing. Since its establishment in December 2023, it has housed diverse groups of animals—including bats, frogs, and turkeys—and has provided scholars and students with a space for research and observation.
This Earth Month, NYU News asked Joachim to tell us more about the principles behind Fab Tree Hab, which is expected to stand for 150-200 years, and the future of sustainable housing.
What sparked this endeavor, and how long did it take to build?
The Fab Tree Hab project, which was initiated at MIT and later became associated with Terreform ONE, was born out of an eco-vision to create truly sustainable and biotech based living spaces. The concept emerged from the desire to rethink traditional architecture and urban planning by integrating living ecosystems into a multi-species environment. The aim was to design homes that were not just in nature, but of nature, using living trees as the primary material to create a living, breathing structure that could coexist harmoniously with its surroundings.
The idea was to use ancient techniques like pleaching—creating a lattice with living and dead branches—intertwining, and weaving, combined with modern fabrication technologies to guide the growth of living trees into the structures of homes. The project started in 2002 and is now growing in a 10-acre lot next to Storm King Art Center in New York.
How does Fab Tree Hab address issues around extinction and sustainability?
The actual time it would take to grow a Fab Tree Hab home would span years, if not decades, due to the nature of growing living trees into a livable structure.
Early model of Fab Tree Hab. Credit: Tracey Friedman
The evolution of the idea and its implementation is an ongoing process, and it seeks to engage biodiversity preservation, carbon sequestration, renewable resources, and reduction in energy. The idea is to offer a holistic solution to sustainability, directly tackling the causes of environmental degradation and species extinction. It represents a radical rethinking of how humans can live in and interact with the natural world, proposing a future where human habitation becomes a contributing factor to ecosystem health rather than a threat.
How will it change with the seasons?
In spring, it will come to life along with the rest of the natural world—buds on the living branches used to construct the home will begin to sprout leaves, gradually filling in the structure with lush greenery. Flowers may bloom on certain parts of the habitation, attracting pollinators and adding color and vibrancy to the structure. By the summer, the Fab Tree Hab will be in full leaf, providing ample shade and a dense, green canopy. This natural foliage will help regulate the interior temperature, keeping it cooler in the heat. The dense leaves will also contribute to the home's privacy and aesthetic appeal. In autumn, the leaves of the home will change color, offering a spectacular display of reds, yellows, and oranges before they fall. This seasonal shedding indicates the structure's direct connection to the cycle of nature, with the fallen leaves decomposing and enriching the soil around the base of the habitation. For the winter, the structure will appear bare and dormant, as deciduous trees lose their leaves, revealing the intricate network of branches that make up its walls and roof. Despite the lack of foliage, the living trees continue to provide structural support. Evergreen plants and structural elements designed to retain their presence year-round could help maintain some degree of greenery and protection against the elements during the colder months.
Microhabitats in the walls of Fab Tree Hab. Credit: Tracey Friedman/New York University
Mitchell Joachim/ Credit: Tracey Friedman
It represents a radical rethinking of how humans can live in and interact with the natural world, proposing a future where human habitation becomes a contributing factor to ecosystem health rather than a threat.
What maintenance is required?
The maintenance required is more akin to gardening than traditional building upkeep. For example, pruning and guiding growth is necessary to shape the growth of the trees according to the structure's design. This involves guiding the trees as they grow and intertwine to maintain the integrity and functionality of the living architecture. It also needs water and nutrients to ensure the trees’ health and growth. The specific requirements would depend on the species of trees used and the local climate. Systems for rainwater capture and distribution might be incorporated into the design to support this.
Like any garden or orchard, the Fab Tree Hab needs monitoring and management to prevent and treat any pest infestations or diseases that could threaten the health of the trees. Additionally, while the structure is living and thus self-healing to an extent, regular checks are essential to ensure that it remains safe and secure as the trees grow and change with time. Finally, the internal environment of a Fab Tree Hab, such as humidity and temperature, is influenced by the living structure, so managing these conditions to ensure a comfortable living space might require innovative solutions.
What kind of animals currently inhabit the space? And how do you expect people to use it?
For now, many creatures have been curious about the space, including deer, raccoons, frogs, butterflies, bats, birds, spiders, wild turkeys, and rabbits. The panel system is made of clusters of more than 60 jute habitats of different sizes and scales. It welcomes all types of organisms from the Hudson Valley region and animals will move in, live, mate, and feed as they choose. We have wildlife cams set up to capture data on their behavior and the panels will adjust to meet the different use models. External groups meant to use it are students to learn about wildlife ecology, artists who are active in the environment, our researchers, and the public.
How do you foresee the future of sustainable housing?
In the near future we can grow homes made from local woody plants, instead of killing trees to sell lumber. In the future, carpenters, contractors, and trade-workers will be trained in arborsmithing and basic landscape ecology. People will be able to recognize the health of trees and integrate them into our built realm.
Mitchel Joachim (far left) and his Fab Tree team at their studio in the Brooklyn Navy Yard, NYC. Credit: Tracey Friedman

Science News by AGU
Animals Deserve to Be Included in Global Carbon Cycle Models, Too
Share this:.
- Click to print (Opens in new window)
- Click to email a link to a friend (Opens in new window)
- Click to share on Twitter (Opens in new window)
- Click to share on Facebook (Opens in new window)
- Click to share on LinkedIn (Opens in new window)

Source: Journal of Geophysical Research: Biogeosciences
Models of the global carbon cycle typically include plants, microbes, soil, and the atmosphere. But they may be leaving out an important variable: Animals, from earthworms to elephants, can have a significant, though heretofore little-studied, influence on how carbon is captured and stored in ecosystems.
A new theoretical framework from Rizzuto et al . offers a road map for including animals in carbon cycle models. Their work shows that adding both herbivores and predators to such models significantly alters both the amount and the dynamics of carbon cycling. Future modeling of carbon dynamics, important for understanding climate change and designing nature-based carbon sequestration projects, should take animals into consideration as well, researchers argue.
Animals affect carbon cycling directly by eating plants or by eating other animals that eat plants. By producing waste, respiring, and even trampling leaves on the forest floor, they also indirectly speed up the rate at which nutrients—including carbon—are recycled. Overall, the authors found, on average, a twofold increase in ecosystem carbon sequestration when animals were included in their carbon cycle model.
By pairing an ecosystem compartment model (which considers plant, animal, and soil microbial trophic compartments) with a traditional carbon modeling approach, the authors found noteworthy increases in both primary productivity (i.e., plant growth) and carbon sequestration, as well as changes to carbon cycle dynamics, when animals are included in carbon models. Importantly, these effects can be complex and are mediated by feedback loops that are still not completely understood. For example, a scenario that includes herbivores but not predators showed the highest levels of carbon sequestration. Adding in predators decreased overall carbon sequestration, though it remained higher than a scenario with no animals.
Adding animals to carbon cycle models leads to a significant “rewiring” of the carbon cycle that will require further study to better understand, the authors conclude. Distinguishing between herbivore and predator types, as well as adding ecosystem-specific animal behavior dynamics to models, could help refine models in the future.
This kind of work could inform future nature-based carbon sequestration proposals and highlights the importance of animals of all kinds for combating climate change. ( Journal of Geophysical Research: Biogeosciences , https://doi.org/10.1029/2024JG008026 , 2024)
—Nathaniel Scharping ( @nathanielscharp ), Science Writer
Citation: Scharping, N. (2024), Animals deserve to be included in global carbon cycle models, too, Eos, 105, https://doi.org/10.1029/2024EO240170 . Published on 16 April 2024.
Text © 2024. agu. cc by-nc-nd 3.0 except where otherwise noted, images are subject to copyright. any reuse without express permission from the copyright owner is prohibited., features from agu publications, lakes worldwide need a checkup, hydraulic fractures are lazy, foundations in hazards and disasters for undergraduate students.

New compound from blessed thistle promotes functional nerve regeneration
Researchers from the University of Cologne have found a new use for Cnicin, a substance produced in blessed thistle.
Blessed thistle (Cnicus benedictus) is a plant in the family Asteraceae and also grows in our climate. For centuries, it has been used as a medicinal herb as an extract or tea, e.g. to aid the digestive system. Researchers at the Center for Pharmacology of University Hospital Cologne and at the Faculty of Medicine of the University of Cologne have now found a completely novel use for Cnicin under the direction of Dr Philipp Gobrecht and Professor Dr Dietmar Fischer. Animal models as well as human cells have shown that Cnicin significantly accelerates axon (nerve fibres) growth. The study 'Cnicin promotes functional nerve regeneration' was published in Phytomedicine .
Rapid help for nerves
Regeneration pathways of injured nerves in humans and animals with long axons are accordingly long. This often makes the healing process lengthy and even frequently irreversible because the axons cannot reach their destination on time. An accelerated regeneration growth rate can, therefore, make a big difference here, ensuring that the fibres reach their original destination on time before irreparable functional deficits can occur. The researchers demonstrated axon regeneration in animal models and human cells taken from retinae donated by patients. Administering a daily dose of Cnicin to mice or rats helped improve paralysis and neuropathy much more quickly.
Compared to other compounds, Cnicin has one crucial advantage: it can be introduced into the bloodstream orally (by mouth). It does not have to be given by injection. "The correct dose is very important here, as Cnicin only works within a specific therapeutic window. Doses that are too low or too high are ineffective. This is why further clinical studies on humans are crucial," said Fischer. The University of Cologne researchers are currently planning relevant studies. The Center for Pharmacology is researching and developing drugs to repair the damaged nervous system.
The current study received funding of around 1,200,000 euros from the Federal Ministry of Education and Research within the framework of the project PARREGERON.
- Brain Injury
- Neuroscience
- Developmental Biology
- Life Sciences
- Biotechnology
- Optic nerve
- Gastrointestinal tract
Story Source:
Materials provided by University of Cologne . Note: Content may be edited for style and length.
Journal Reference :
- Philipp Gobrecht, Jeannette Gebel, Marco Leibinger, Charlotte Zeitler, Zhendong Chen, Dirk Gründemann, Dietmar Fischer. Cnicin promotes functional nerve regeneration . Phytomedicine , 2024; 155641 DOI: 10.1016/j.phymed.2024.155641
Cite This Page :
Explore More
- This Alloy Is Kinky
- Giant Galactic Explosion: Galaxy Pollution
- Flare Erupting Around a Black Hole
- Two Species Interbreeding Created New Butterfly
- Warming Antarctic Deep-Sea and Sea Level Rise
- Octopus Inspires New Suction Mechanism for ...
- Cities Sinking: Urban Populations at Risk
- Puzzle Solved About Ancient Galaxy
- How 3D Printers Can Give Robots a Soft Touch
- Combo of Multiple Health Stressors Harming Bees
Trending Topics
Strange & offbeat.

- April 22, 2024 | Chasing Martian Mysteries: Perseverance Pays Off When Studying the Red Planet’s Atmosphere
- April 22, 2024 | Scientists Discover New Antibiotic Class Effective Against Drug-Resistant Bacteria
- April 22, 2024 | Challenging Historical Interpretations: Scientists Shed New Light on the Mysterious Cerne Giant
- April 22, 2024 | Vampire Bacteria? Scientists Uncover Blood-Hunting Behavior in Common Bacteria
- April 22, 2024 | Astronomers Discover 49 New Galaxies in Under Three Hours
New Research Reveals That Some Plant-Based Steaks and Cold Cuts Are Lacking in Protein
By American Chemical Society April 21, 2024
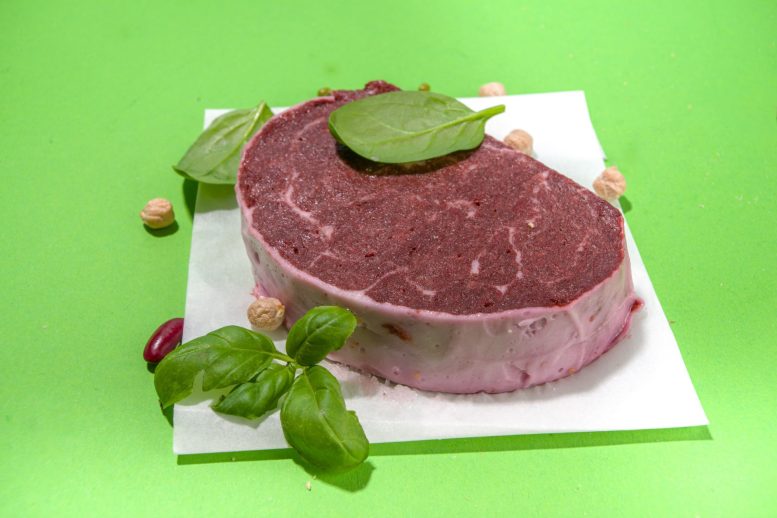
A recent study indicates that plant-based meats, while similar in taste and texture to animal products, generally contain more carbohydrates and less protein, with varying levels of amino acids and protein digestibility. The research suggests the need for greater consumer awareness of these nutritional differences.
Plant-based meats have impressively mimicked a range of animal products, from beef to seafood. However, the question remains: how do they stack up nutritionally? According to a recent study in the Journal of Agricultural and Food Chemistry published by ACS, while some plant-based alternatives like “plant steaks” and “plant cold cuts” may rival real meats in certain aspects, they fall short in areas such as amino acid content and protein digestibility.
Meat-free burgers or ground beef mimics might come to mind first, but the options for plant-based alternatives have expanded to include whole cuts of meat resembling steaks and chicken breasts, as well as sliced cold cuts like salami or bresaola — a type of cured beef. While these newer products haven’t been studied as extensively as burger-style products, they are becoming more widespread and popular among consumers.
As a result, it’s important to understand how they differ nutritionally from the meats they aim to replicate and replace. In other words, how well do our bodies digest and gain nutrition from these foods? Tullia Tedeschi and colleagues wanted to answer that question by comparing the protein quality, integrity, and digestibility of a set of plant-based steaks and cold cuts to their meat counterparts.
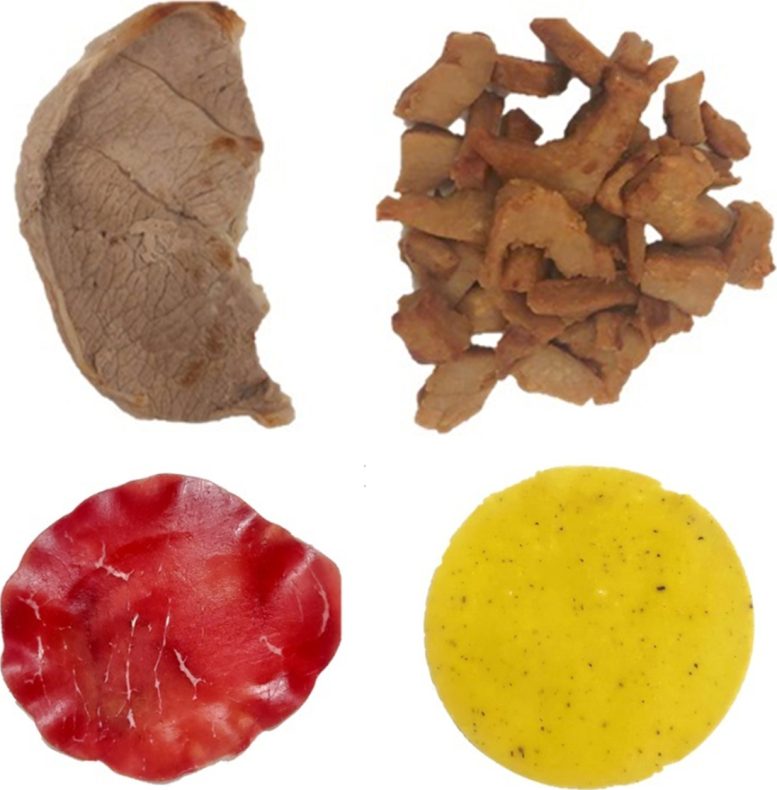
Meat products (top left, veal; bottom left, bresaola) tend to contain more proteins and amino acids than their plant-based alternatives (right). Credit: Adapted from Journal of Agricultural and Food Chemistry 2024, DOI: 10.1021/acs.jafc.3c08956
Methodology and Initial Findings
The team, based in Italy, collected three different plant-based steaks and three different plant-based cold cuts. Veal steaks were used as a comparison point for the plant steaks, whereas ham and beef cold cuts were compared to their respective plant-based substitutes. The fat, salt and protein content of each was measured, then the samples underwent a simulated digestion in the lab to understand how well the proteins break down in a human’s digestive tract.
- The plant-based products contained more carbohydrates, less protein, and reduced amino acid content than their meat-based counterparts.
- Plant steaks and the veal samples were comparable in terms of essential amino acid content and digestibility.
- Plant cold cuts generally had less salt than the meats and contained fewer essential amino acids . Different products also showed differing levels of digestibility due to the variety of ingredients they contain.
Overall, the nutritional value of the plant-based products depended greatly on the plants used to create them, causing wide variation in their amino acid content and the digestibility of their proteins. In contrast, all the samples within a particular meat type showed comparable nutritional profiles. The researchers say that this work helps demonstrate that careful consideration should be taken when replacing meat products with plant-based alternatives, and that these differences in nutritional profile should be communicated to consumers to allow for informed decisions.
Reference: “Assessment of Protein Quality and Digestibility in Plant-Based Meat Analogues” by Sara Cutroneo, Barbara Prandi, Nicoletta Pellegrini, Stefano Sforza and Tullia Tedeschi, 1 April 2024, Journal of Agricultural and Food Chemistry . DOI: 10.1021/acs.jafc.3c08956
The authors acknowledge funding from the Emilia Romagna Region of Italy for this work.
More on SciTechDaily
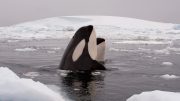
Certain Female Whales Can Live up to 40 Years Longer – Scientists Have Finally Discovered the Secret
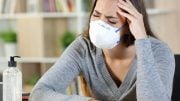
Long COVID: Lasting Effects for the 1 in 10 COVID-19 Patients Who Become Long-Haulers
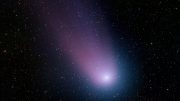
Trajectory Models of Refractory Particles Help Solve Two Solar System Puzzles
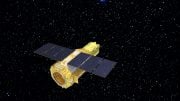
X-Ray Vision of the Universe: XRISM Spacecraft Will Open New Window on the Cosmos
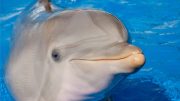
Scientists Discover New Sense of Bottlenose Dolphins: They Feel Electricity
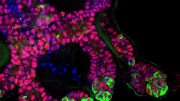
Growing Human Kidneys in a Lab – Scientists Have Made a Significant Breakthrough
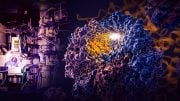
Profound Implications: New Research Challenges a 70-Year-Old Theory of Protein Folding
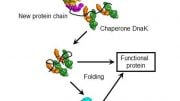
DnaK Identified as Key Player of Protein Folding
1 comment on "new research reveals that some plant-based steaks and cold cuts are lacking in protein".
“The poisons will be hidden in everything that surrounds them, in what they drink, eat, breathe and wear.”
https://ia804602.us.archive.org/4/items/tsc_7/TSC.pdf
Leave a comment Cancel reply
Email address is optional. If provided, your email will not be published or shared.
Save my name, email, and website in this browser for the next time I comment.
- Collections
- Recent Additions
- Submission Information
- Content and Copyright
- home NOAA IR Home
Plant and Animal Habitats (Science Grade 2 Lesson Plan/Activity)

Search our Collections & Repository
- Advanced Search
- Custom Query
All these words:
For very narrow results
This exact word or phrase:
When looking for a specific result
Any of these words:
Best used for discovery & interchangable words
None of these words:
Recommended to be used in conjunction with other fields
Publication Date Range:
Document Type:
Collection:
Query Builder
For additional assistance using the Custom Query please check out our Help Page
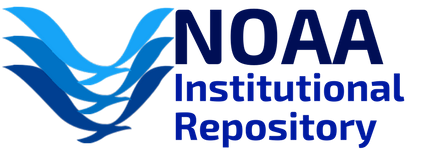
- By Simpkins, Grace ; Irving, Michael
- Personal Author: Simpkins, Grace ; Irving, Michael Simpkins, Grace ; Irving, Michael Less -
- NOAA Program & Office: OAR (Oceanic and Atmospheric Research) ; Sea Grant OAR (Oceanic and Atmospheric Research) ; Sea Grant Less -
- Sea Grant Program: WHOI (Woods Hole Sea Grant)
- Description: In this 2nd grade unit, the students will explore the habitats of plants and animals. A habitat is a place where an animal or plant lives that meets its need to survive. Students will learn that animals must have oxygen, food/water, and shelter. Students will learn that plants need carbon dioxide, sun, water, and food (minerals). Students will also observe adaptations that allow animals and plants to avoid predators. Finally, the students will learn how plants and animals rely on each other to survive and reproduce. Included are the digital for the lesson plan, observation sheet, Venn Diagram, Human habitat worksheet, Marine Animals I have seen worksheet, Plant observation worksheet, and Master unit vocabulary list. More ▼ -->
- Keywords: [+] Lesson plan
- Sea Grant Document Number: WHOI-E-20-003
- Document Type: Instructional Material
- Funding: Grant no. NA18OAR4170104
- Rights Information: Public Domain
- Compliance: Library
- Main Document Checksum: [+] urn:sha256:825d86c67187d6af6527dc86fb25d608c83d53c0d8f535e3375de8e41f0bad3c
- Download URL: https://repository.library.noaa.gov/view/noaa/39080/noaa_39080_DS1.pdf
Supporting Files
You may also like.
Checkout today's featured content at repository.library.noaa.gov
Exit Notification/Disclaimer Policy
- The National Oceanic and Atmospheric Administration (NOAA) cannot attest to the accuracy of a non-federal website.
- Linking to a non-federal Website does not constitute an endorsement by NOAA or any of its employees of the sponsors or the information and products presented on the website.
- You will be subject to the destination website's privacy policy when you follow the link.
- NOAA is not responsible for Section 508 compliance (accessibility) on other federal or private websites.
What to do when you encounter a baby animal in the wild
MINOCQUA, Wis. (WSAW) - We’re now in the time of year when wild animals are having their babies and that means their safety, and yours, is important. Especially if you find a baby animal abandoned in your area.
If you come across an abandoned baby animal, make sure it’s safe to approach it. You should then call your nearby rehabilitation center. The Northwoods Wildlife Center in Minocqua sees plenty of small animals, but their most common is the eastern gray squirrel.
“Once an animal does come in, however, if it’s injured, or in some cases orphaned, then my role as a wildlife rehabilitator is to help raise that animal if it is an infant,” Northwoods Wildlife Center Wildlife Rehabilitator Sheridan Perry said.
Perry says they encourage people to try and approach the animal if they can to see what their behavior is like.
“Is it lying there, quiet and lethargic, maybe on its side, breathing heavily? Or, is it active and alert, and runs away from the person when they approach it?” she said.
If it’s possible, people are encouraged to reunite the baby animals with their parents.
“Birds absolutely will take care of their babies if you handle them,” Perry said. “So, if you see a nest laying on the ground, picking it up and putting it back in its nest, if you’re able to find it, is fine.”
People might also assume that Eastern cotton tail rabbits aren’t old enough to be on their own — that’s not the case.
“If you see a baby cotton-tailed rabbit and it’s about the size of a tennis ball, it’s actually old enough to be on its own and doesn’t need to come into a center at all unless it’s injured,” Perry said.
When you call your wildlife center, they might tell you to get the animal safely in a crate or a box.
“That could be a cardboard box, an empty dog crate, something like that,” Perry added. “Get it to a warm, quiet, dark location until they’re able to bring it into the center.”
Perry says taking pictures of the animal will also help them determine the species and the severity of any injury or illness the animal may have.
Copyright 2024 WSAW. All rights reserved.
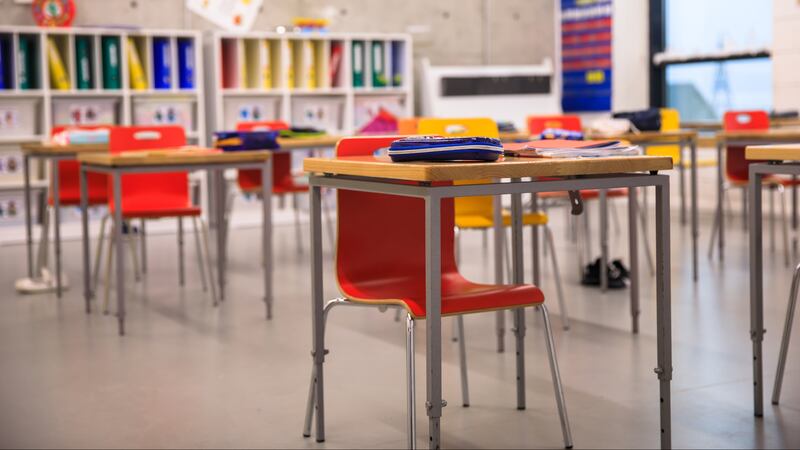
School district cancels lesson on pronouns after staff receives threats

Concerning level of pesticides found in frozen and non organic fruits and vegetables imported into US

Police officer fatally shot overnight while heading home from work

1 injured in car vs. motorcycle crash in Ashland County

Stratford native Macey Kilty falls in U.S. Olympic Team Wrestling Trials finals
Latest news.

Pittsville, Port Edwards softball unite to honor life of Pittsville player

Highlights: UW-Stevens Point softball moves to top of standings with sweep of UW-Platteville

You Know You’re From...Rhinelander: Working around the clock
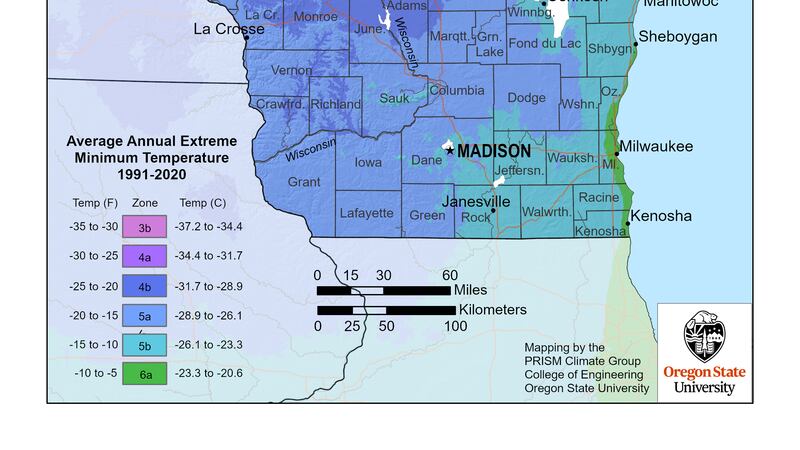
Gardeners who’ve taken a risk to plant could see payoffs grow this spring and summer
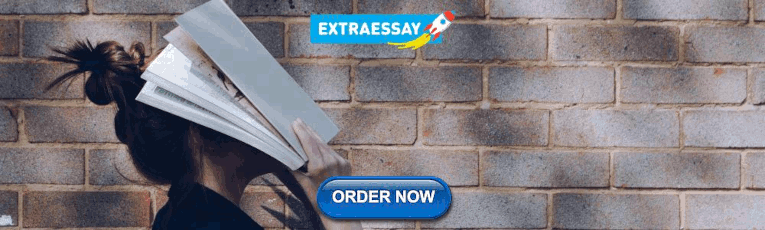
IMAGES
VIDEO
COMMENTS
Plant sciences is the study of plants in all their forms and interactions using a scientific approach. Featured Deciphering the mechanisms of gene silencing induced by triplet-repeat expansions
Plant-animal interaction affects restoration. Ecological restoration and conservation are essential to protecting the environment ( 1 ). Recent efforts have focused on the physical landscape, such as planting as many trees as possible ( 2 ). However, tree planting will not achieve the desired results if interactions between plants and animals ...
Field Guide to Brazil (1960) Browse 168 science publications on Biology and Life Sciences - Animals, Plants and Other Organisms from the National Academies Press.
Perhaps the Krogh Principle needs revision; as written, plants (and, for the matter, members of all kingdoms) are "animals" too. But the interdependence of plant and animal studies is even more fundamental. As the most elementary of biology teaches, most plants and animals rely on one another for energy and mass fixation, and/or reproduction.
Wandersee and Schussler (1999, 2001), drawing on visual cognition research, introduced the idea of plant blindness. ... were asked to perform a dual-target task in which they were to report whether or not they saw an image of a plant/animal (target T1) and whether or not they saw an image of water (target T2). Both T1 and T2 could be present or ...
Plants make our existence possible. All of the articles featured in this special issue, including this one, were first presented at a National Geographic Society symposium entitled "A World of Plants" 29-30 October 2019, in Washington, DC, USA. The presented topics were selected to provide a wide range of information on plants and ...
Structural Biology: A Gateway to Understanding Metabolic and Signaling Pathways in Plants. Milosz Ruszkowski. Bartosz Sekula. ISABEL NOGUES. 992 views. 1 article. The most cited plant science journal advances our understanding of plant biology for sustainable food security, functional ecosystems and human health.
Since the seminal book On the Origin of Species by Charles Darwin, modern ecologists have been aware that the origin, maintenance and function of biodiversity are products of biotic interactions among species (Darwin 1859; Ings and Hawes 2018).Particularly, the majority of animal and plant species are embedded in highly diverse trophic systems which include different types of organisms within ...
Plant biology is a key area of science that bears major weight in the mankind's ongoing and future efforts to combat the consequences of global warming, climate change, pollution, and population growth. An in-depth understanding of plant physiology is paramount to our ability to optimize current agricultural practices, to develop new crop ...
Plant physiology is a sub-discipline of botany concerned with the physical, chemical and biological functioning of plants. Latest Research and Reviews Measurement of mass force field driving water ...
The main difference between animals and plants is that the ... Scientists from no less than thirty-three institutions working in plant research claimed that plant neurobiology was based only on superficial analogies and dubious extrapolations that added nothing to the understanding of plant biology. 31 It is notable that the title of ...
Small RNAs (microRNAs, siRNAs, piRNAs and others) function as agents of intercellular communication, particularly in development, reproduction, immunity and inheritance. Chen and Rechavi discuss ...
Trees can outsmart animals such as squirrels and birds by synchronizing their seed production. Emily Moran, The Conversation US. Genetics October 26, 2023. ... Plants June 19, 2023.
Plant-Animal Interactions: Exploring Costs and Benefits in Highly Conditional Relationships ... For planned papers, a title and short abstract (about 100 words) can be sent to the Editorial Office for announcement on this website. ... By revealing the protective function of spiders' vegetative structures on plants, this research highlights ...
Research on plant systems is expanding, with implications for human health and ecosystem services—from climate change and bioenergy to drug discovery and food science. Plants do many interesting things at multiple scales that include, to mention a few, complex secondary metabolism, gene regulation, genomic evolution (while animals simply move ...
The present paper will review the use of plants and their extracts to manipulate the rumen microbial ecosystem to improve the efficiency of rumen metabolism. The bioavailability of secondary metabolites and their actions on peripheral metabolism will be considered with a view to improving animal performance. The challenge of delivering plants ...
Fig. 1 shows data from Heller and Keoleian's (2018) analysis of Beyond meat compared to conventional beef, but such magnitudes of disparity are typical across other similar analyses (see Section 5).As shown, the environmental impact of PB-APAs relative to their animal-based counterparts is close to zero. That is to say, even if whole plant foods had no environmental impact whatsoever (which is ...
A male plant or animal contributes genetic material in the form of sperm or pollen to a female plant or animal's egg. The offspring then has genetic material from both parents. Different plants and animals can reproduce either asexually or sexually; however, a sexual reproduction is more common among plants than animals.
Plants and animals share many characteristics, but they are different in some respects. Animals usually move around and find their own food, while plants are usually immobile and create their food via photosynthesis. Plants and animals both have cells that contain DNA, yet the structure of their cells differs. Animal cells absorb nutrients from ...
Plant Matter. The material presences of plants in writing make possible a play of sign and referent from literature's inception. Carved with a blunt reed, the remaining fragments of the oldest literary text, The Epic of Gilgamesh (c. 2100 bce), include a story of the hero's discovery and loss of a plant that would bring immortality.From ancient times, the plant matter of literary musing ...
Plants and animals are classified using the Linnaean taxonomy. It is a hierarchy system that organizes living things into groups based upon common features. The system operates on a 8-step cascading hierarchy that branches off from the top category ('domain'), which separates all living things into three buckets, down to 'species ...
mountain ecosystem, complex of living organisms in mountainous areas. Mountain lands provide a scattered but diverse array of habitats in which a large range of plants and animals can be found. At higher altitudes harsh environmental conditions generally prevail, and a treeless alpine vegetation, upon which the present account is focused, is ...
Various groups including animal protection organizations, medical organizations, research centers, and even federal agencies such as the U.S. Food and Drug Administration, are working to minimize animal use in scientific experiments. This movement primarily stems from animal welfare and ethical concerns. However, recent advances in technology and new studies in medicine have contributed to an ...
A Habitat for Humanity—And Plants and Animals, Too. A team led by Gallatin Professor Mitchell Joachim created a 'coral reef' for land that may usher in a new era of sustainable housing. Goodbye, brick and mortar. Hello, wood and jute! As we try to imagine the future of sustainable architecture, an innovative structure in New Windsor, NY ...
Models of the global carbon cycle typically include plants, microbes, soil, and the atmosphere. But they may be leaving out an important variable: Animals, from earthworms to elephants, can have a ...
Aug. 28, 2023 — Scientists have verified the anticancer effects of Kencur, a tropical plant of the ginger family, mainly grown in Southeast Asia, in cell and animal experiments. They found that ...
According to a recent study in the Journal of Agricultural and Food Chemistry published by ACS, while some plant-based alternatives like "plant steaks" and "plant cold cuts" may rival real meats in certain aspects, they fall short in areas such as amino acid content and protein digestibility. Meat-free burgers or ground beef mimics ...
Collaboration supports student enrichment and workforce development in North Carolina. EAST GREENSBORO, N.C. (April 19, 2024) - Merck (NYSE: MRK), known as MSD outside of the United States and Canada, and North Carolina Agricultural and Technical State University announced today the opening of the Merck Biotechnology Learning Center at Gateway Research Park in Greensboro, North Carolina.
In this 2nd grade unit, the students will explore the habitats of plants and animals. A habitat is a place where an animal or plant lives that meets its need to survive. Students will learn that animals must have oxygen, food/water, and shelter. Students will learn that plants need carbon dioxide, sun, water, and food (minerals).
If it's possible, people are encouraged to reunite the baby animals with their parents. "Birds absolutely will take care of their babies if you handle them," Perry said. "So, if you see a ...