Pharmaceutical Industry, Its History and Future Essay
History of the industry.
The pharmaceutical industry is a relatively new industry, the origins of which can be traced to the mid-19th century. Until that time, there was no mass production of drugs; apothecaries relied mainly on natural remedies, which were ineffective in solving the most prevalent health issues of the time. The emergence of the pharmaceutical industry was largely associated with the overall development of science and medicine. The scientific developments related to the creation and application of drugs such as morphine and strychnine has led to the need for mass production of these drugs. This, in turn, caused apothecaries to initiate wholesale production and distribution, abandoning the previous direct sale channels. Similarly, well-established chemical companies also moved into the emerging market of chemical drugs, after researchers discovered an application for their chemicals in medicine and science.
Pharmaceutical firms first emerged in Germany at the end of the 19th century, but soon gained popularity in other areas of the globe, such as England and America. The pharmaceutical industry grew in pace with the growth of medical technology, expanding the range of products available. However, the development of new drugs required a lot of time and effort, making it difficult for individual scientists or small groups to search for new medicine. As the popularity and income of the pharmaceutical industry grew, pharmaceutical firms began forming partnerships with laboratories and research groups in order to expedite the development of new products and the improvement of existing ones. These partnerships continue today, with most pharmaceutical companies owning state-of-the-art laboratories, staffed with highly qualified research groups. Apart from the advancement of medical science, the pharmaceutical industry was also influenced by government regulations. For instance, in some countries, pharmaceutical companies were able to form stable partnerships with the government, thus achieving stability and increasing their market share.
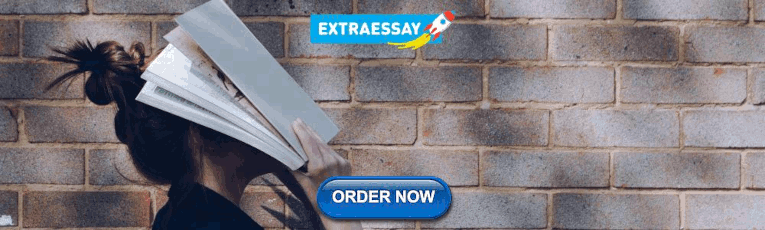
Predict the Future
Despite the significant progress in medical research, the number of health issues faced by people all over the world is still high, which is why it is likely that the pharmaceutical industry will continue to grow in the future. However, as identified in the previous section, there are some serious threats to the industry that the future innovators will have to resolve. In order for the industry to move forward, it is crucial to focus on solving the health issues that are currently perceived by people as the most pressing, such as cancer, obesity, and cardiovascular diseases. In order to promote innovation and ensure further growth of the industry, it is crucial for the industry leaders to respond to the identified threats by:
- establishing partnerships across the industry to achieve a competitive advantage;
- reducing the costs by using efficient technologies and effective HR practices, including talent management;
- increasing the range of products to compete with Little Pharma and generic companies;
- building stronger community connections to appeal to customers (for instance, by holding health education events, fundraisers, etc.);
- reviewing and updating regulatory policies to reflect any external changes.
The innovators in the pharmaceutical industry will most likely arise in Big Pharma companies, as they are under significant pressure to develop new strategies. If companies manage to build strong connections with partners, communities, and the government, they will be able to ensure the future success of the entire industry by solving many of the current challenges, including the increased competitive pressure, changes to the regulations, and more.
- Chicago (A-D)
- Chicago (N-B)
IvyPanda. (2021, January 10). Pharmaceutical Industry, Its History and Future. https://ivypanda.com/essays/pharmaceutical-industry-its-history-and-future/
"Pharmaceutical Industry, Its History and Future." IvyPanda , 10 Jan. 2021, ivypanda.com/essays/pharmaceutical-industry-its-history-and-future/.
IvyPanda . (2021) 'Pharmaceutical Industry, Its History and Future'. 10 January.
IvyPanda . 2021. "Pharmaceutical Industry, Its History and Future." January 10, 2021. https://ivypanda.com/essays/pharmaceutical-industry-its-history-and-future/.
1. IvyPanda . "Pharmaceutical Industry, Its History and Future." January 10, 2021. https://ivypanda.com/essays/pharmaceutical-industry-its-history-and-future/.
Bibliography
IvyPanda . "Pharmaceutical Industry, Its History and Future." January 10, 2021. https://ivypanda.com/essays/pharmaceutical-industry-its-history-and-future/.
- Painkillers Distribution and Its Consequences
- Legal and Ethical Scenarios of Decision Making
- Corporate Strategic Marketing: Analyzing Various Markets
- Interventions to Improve Quality of Care
- Amphetamines and Their Effects on Memory
- Levocarnitine: Properties and Application
- Tricyclic Antidepressants for Back Pain Treatment
- Advanced Pharmacology: Nurse Practitioner Prescribing

Refine Results By
Research and development in the pharmaceutical industry.

At a Glance
This report examines research and development (R&D) by the pharmaceutical industry.
Spending on R&D and Its Results. Spending on R&D and the introduction of new drugs have both increased in the past two decades.
- In 2019, the pharmaceutical industry spent $83 billion dollars on R&D. Adjusted for inflation, that amount is about 10 times what the industry spent per year in the 1980s.
- Between 2010 and 2019, the number of new drugs approved for sale increased by 60 percent compared with the previous decade, with a peak of 59 new drugs approved in 2018.
Factors Influencing R&D Spending. The amount of money that drug companies devote to R&D is determined by the amount of revenue they expect to earn from a new drug, the expected cost of developing that drug, and policies that influence the supply of and demand for drugs.
- The expected lifetime global revenues of a new drug depends on the prices that companies expect to charge for the drug in different markets around the world, the volume of sales they anticipate at those prices, and the likelihood the drug-development effort will succeed.
- The expected cost to develop a new drug—including capital costs and expenditures on drugs that fail to reach the market—has been estimated to range from less than $1 billion to more than $2 billion.
- The federal government influences the amount of private spending on R&D through programs (such as Medicare) that increase the demand for prescription drugs, through policies (such as spending for basic research and regulations on what must be demonstrated in clinical trials) that affect the supply of new drugs, and through policies (such as recommendations for vaccines) that affect both supply and demand.
To remove the effects of inflation, the Congressional Budget Office adjusted dollar amounts with the gross domestic product price index from the Bureau of Economic Analysis. Amounts are expressed in 2019 dollars.
Every year, the U.S. pharmaceutical industry develops a variety of new drugs that provide valuable medical benefits. Many of those drugs are expensive and contribute to rising health care costs for the private sector and the federal government. Policymakers have considered policies that would lower drug prices and reduce federal drug expenditures. Such policies would probably reduce the industry’s incentive to develop new drugs.
In this report, the Congressional Budget Office assesses trends in spending for drug research and development (R&D) and the introduction of new drugs. CBO also examines factors that determine how much drug companies spend on R&D: expected global revenues from a new drug; cost to develop a new drug; and federal policies that affect the demand for drug therapies, the supply of new drugs, or both.
What Are Recent Trends in Pharmaceutical R&D and New Drug Approvals?
The pharmaceutical industry devoted $83 billion to R&D expenditures in 2019. Those expenditures covered a variety of activities, including discovering and testing new drugs, developing incremental innovations such as product extensions, and clinical testing for safety-monitoring or marketing purposes. That amount is about 10 times what the industry spent per year in the 1980s, after adjusting for the effects of inflation. The share of revenues that drug companies devote to R&D has also grown: On average, pharmaceutical companies spent about one-quarter of their revenues (net of expenses and buyer rebates) on R&D expenses in 2019, which is almost twice as large a share of revenues as they spent in 2000. That revenue share is larger than that for other knowledge-based industries, such as semiconductors, technology hardware, and software.
The number of new drugs approved each year has also grown over the past decade. On average, the Food and Drug Administration (FDA) approved 38 new drugs per year from 2010 through 2019 (with a peak of 59 in 2018), which is 60 percent more than the yearly average over the previous decade.
Many of the drugs that have been approved in recent years are “specialty drugs.” Specialty drugs generally treat chronic, complex, or rare conditions, and they may also require special handling or monitoring of patients. Many specialty drugs are biologics (large-molecule drugs based on living cell lines), which are costly to develop, hard to imitate, and frequently have high prices. Previously, most drugs were small-molecule drugs based on chemical compounds. Even while they were under patent, those drugs had lower prices than recent specialty drugs have. Information about the kinds of drugs in current clinical trials indicates that much of the industry’s innovative activity is focused on specialty drugs that would provide new cancer therapies and treatments for nervous-system disorders, such as Alzheimer’s disease and Parkinson’s disease.
What Factors Influence Spending for R&D?
Drug companies’ R&D spending decisions depend on three main factors:
- Anticipated lifetime global revenues from a new drug,
- Expected costs to develop a new drug, and
- Policies and programs that influence the supply of and demand for prescription drugs.
Various considerations inform companies’ expectations about a drug’s revenue stream, including the anticipated prices it could command in different markets around the world and the expected global sales volume at those prices (given the number of people who might use the drug). The prices and sales volumes of existing drugs provide information about consumers’ and insurance plans’ willingness to pay for drug treatments. Importantly, when drug companies set the prices of a new drug, they do so to maximize future revenues net of manufacturing and distribution costs. A drug’s sunk R&D costs—that is, the costs already incurred in developing that drug—do not influence its price.
Developing new drugs is a costly and uncertain process, and many potential drugs never make it to market. Only about 12 percent of drugs entering clinical trials are ultimately approved for introduction by the FDA. In recent studies, estimates of the average R&D cost per new drug range from less than $1 billion to more than $2 billion per drug. Those estimates include the costs of both laboratory research and clinical trials of successful new drugs as well as expenditures on drugs that do not make it past the laboratory-development stage, that enter clinical trials but fail in those trials or are withdrawn by the drugmaker for business reasons, or that are not approved by the FDA. Those estimates also include the company’s capital costs—the value of other forgone investments—incurred during the R&D process. Such costs can make up a substantial share of the average total cost of developing a new drug. The development process often takes a decade or more, and during that time the company does not receive a financial return on its investment in developing that drug.
The federal government affects R&D decisions in three ways. First, it increases demand for prescription drugs, which encourages new drug development, by fully or partially subsidizing the purchase of prescription drugs through a variety of federal programs (including Medicare and Medicaid) and by providing tax preferences for employment-based health insurance.
Second, the federal government increases the supply of new drugs. It funds basic biomedical research that provides a scientific foundation for the development of new drugs by private industry. Additionally, tax credits—both those available to all types of companies and those available to drug companies for developing treatments of uncommon diseases—provide incentives to invest in R&D. Similarly, deductions for R&D investment can be used to reduce tax liabilities immediately rather than over the life of that investment. Finally, the patent system and certain statutory provisions that delay FDA approval of generic drugs provide pharmaceutical companies with a period of market exclusivity, when competition is legally restricted. During that time, they can maintain higher prices on a patented product than they otherwise could, which makes new drugs more profitable and thereby increases drug companies’ incentives to invest in R&D.
Third, some federal policies affect the number of new drugs by influencing both demand and supply . For example, federal recommendations for specific vaccines increase the demand for those vaccines and provide an incentive for drug companies to develop new ones. Additionally, federal regulatory policies that influence returns on drug R&D can bring about increases or decreases in both the supply of and demand for new drugs.
Trends in R&D Spending and New Drug Development
Private spending on pharmaceutical R&D and the approval of new drugs have both increased markedly in recent years, resuming a decades-long trend that was interrupted in 2008 as generic versions of some top-selling drugs became available and as the 2007–2009 recession occurred. In particular, spending on drug R&D increased by nearly 50 percent between 2015 and 2019. Many of the drugs approved in recent years are high-priced specialty drugs for relatively small numbers of potential patients. By contrast, the top-selling drugs of the 1990s were lower-cost drugs with large patient populations.
R&D Spending
R&D spending in the pharmaceutical industry covers a variety of activities, including the following:
- Invention , or research and discovery of new drugs;
- Development , or clinical testing, preparation and submission of applications for FDA approval, and design of production processes for new drugs;
- Incremental innovation , including the development of new dosages and delivery mechanisms for existing drugs and the testing of those drugs for additional indications;
- Product differentiation , or the clinical testing of a new drug against an existing rival drug to show that the new drug is superior; and
- Safety monitoring , or clinical trials (conducted after a drug has reached the market) that the FDA may require to detect side effects that may not have been observed in shorter trials when the drug was in development.
In real terms, private investment in drug R&D among member firms of the Pharmaceutical Research and Manufacturers of America (PhRMA), an industry trade association, was about $83 billion in 2019, up from about $5 billion in 1980 and $38 billion in 2000 . 1 Although those spending totals do not include spending by many smaller drug companies that do not belong to PhRMA, the trend is broadly representative of R&D spending by the industry as a whole. 2 A survey of all U.S. pharmaceutical R&D spending (including that of smaller firms) by the National Science Foundation (NSF) reveals similar trends. 3
Although total R&D spending by all drug companies has trended upward, small and large firms generally focus on different R&D activities. Small companies not in PhRMA devote a greater share of their research to developing and testing new drugs, many of which are ultimately sold to larger firms (see Box 1 ). By contrast, a greater portion of the R&D spending of larger drug companies (including those in PhRMA) is devoted to conducting clinical trials, developing incremental “line extension” improvements (such as new dosages or delivery systems, or new combinations of two or more existing drugs), and conducting postapproval testing for safety-monitoring or marketing purposes.
Large and Small Drug Companies and the “Make or Buy” Decision
Small drug companies (those with annual revenues of less than $500 million) now account for more than 70 percent of the nearly 3,000 drugs in phase III clinical trials. 1 They are also responsible for a growing share of drugs already on the market: Since 2009, about one-third of the new drugs approved by the Food and Drug Administration have been developed by pharmaceutical firms with annual revenues of less than $100 million. 2 Large drug companies (those with annual revenues of $1 billion or more) still account for more than half of new drugs approved since 2009 and an even greater share of revenues, but they have only initiated about 20 percent of drugs currently in phase III clinical trials. 3
For a large drug company, one option for increasing the number of drugs it expects to introduce is to acquire a smaller firm that is developing new drugs. Over the past three decades, about one-fifth of drugs in development—or the companies developing them—have been acquired by another pharmaceutical company. 4
When a large company acquires a small drug company or the rights to one of its drugs, it can use its specialized knowledge to increase the value of its acquisition or to diversify its risk of a decline in revenues (from a drug’s loss of patent protection, for instance). In making that acquisition, a large company might bring a drug to market more quickly than the small company could have or might distribute it more widely. With the rise of generic drugs, the loss in sales revenues that occurs when a drug’s patent expires can leave firms with excess capacity in production. Acquiring a smaller company can help quickly fill that capacity.
The acquisition of a small company by a larger one can create efficiencies that might increase the combined value of the firms by allowing drug companies of different sizes—in terms of the number of researchers, administrative employees, and financial and physical assets—to specialize in activities in which they have a comparative advantage. Small companies—with relatively fewer administrative staff, less expertise in conducting clinical trials, and less physical and financial capital to manage—can concentrate primarily on research. For their part, large drug companies are much better capitalized and can more easily finance and manage clinical trials. They also have readier access to markets through established drug distribution networks and relationships with buyers.
Researchers have found some evidence that such acquisitions by larger drug firms are sometimes motivated by large firms’ desire to limit competition. According to a recent study of acquisitions in the pharmaceutical industry, for example, a company was about 5 percent to 7 percent less likely to complete the development of drugs in its acquired company’s pipeline if those drugs would compete with the acquirer’s existing drugs than it would be otherwise. 5 In a 2017 study of competition and research and development (R&D), the Government Accountability Office cited several retrospective studies of mergers in the drug industry that found such transactions reduced R&D spending and patenting for several years. 6 The reverse was also true: Increases in pharmaceutical industry competition have been found to increase firms’ R&D spending. 7
1 . See IQVIA Institute for Human Data Science, The Changing Landscape of Research and Development (April 2019), p. 15, https://tinyurl.com/1cm3g2fs .
2 . See Ulrich Geilinger and Chandra Leo, HBM New Drug Approval Report (HBM Partners, January 2019), p. 16. https://tinyurl.com/yyzze476 , (PDF, 1.14 MB). HBM Partners is a Swiss health care investment company.
3 . The 30 largest companies have developed 53 percent of drugs approved since 2009, and in 2014, the 25 largest drug companies received more than 70 percent of industry revenues. See IQVIA Institute for Human Data Science, The Changing Landscape of Research and Development (April 2019), p. 16, https://tinyurl.com/y2kpxve8 ; and Government Accountability Office, Drug Industry: Profits, Research and Development Spending, and Merger and Acquisition Deals , GAO-18-40 (November 2017), p. 16, www.gao.gov/products/GAO-18-40 .
4 . See Colleen Cunningham, Florian Ederer, and Song Ma, “Killer Acquisitions,” Journal of Political Economy , vol. 129, no. 3 (March 2021), p. 670, http://dx.doi.org/10. 1086/712506 .
5 . Ibid., pp. 649–702.
6 . See Government Accountability Office, Drug Industry: Profits, Research and Development Spending, and Merger and Acquisition Deals , GAO-18-40 (November 2017), p. 16, www.gao.gov/products/GAO-18-40 . For the individual studies, see Carmine Ornaghi, “Mergers and Innovation in Big Pharma,” International Journal of Industrial Organization , vol. 27, no. 1 (January 2009), pp. 70–79, https://doi.org/10.1016/j.ijindorg.2008.04.003 ; and Patricia M. Danzon, Andrew Epstein, and Sean Nicholson, “Mergers and Acquisitions in the Pharmaceutical and Biotech Industries,” Managerial and Decision Economics , vol. 28, no. 4/5 (June–August 2007), pp. 307–328, www.jstor.org/stable/25151520 .
7 . See Richard T. Thakor and Andrew W. Lo, “Competition and R&D Financing: Evidence From the Biopharmaceutical Industry,” Journal of Financial and Quantitative Analysis (forthcoming), http://dx.doi.org/10.2139/ssrn.3754494 .
CBO relied on the PhRMA data because before 2008, the NSF survey did not include domestic firms’ R&D spending outside of the United States. (Both the NSF and PhRMA estimates reflect worldwide R&D spending by pharmaceutical companies with operations in the United States.) NSF’s estimates of R&D spending since 2008 suggest that PhRMA members’ worldwide R&D spending constitutes about 75 percent to 85 percent of the industry total, depending on the year.
In recent years, the pharmaceutical industry’s R&D spending as a share of net revenues (sales less expenses and rebates) has increased: Consumer spending on brand-name prescription drugs has risen, but R&D spending has risen more quickly. In the early 2000s, when drug industry revenues were rising sharply, the industry’s R&D intensity—that is, its R&D spending as a share of net revenues—averaged about 13 percent each year. Over the decade from 2005 to 2014, the industry’s R&D intensity averaged 18 percent to 20 percent each year. That ratio has been trending upward since 2012, and it exceeded 25 percent in 2018 and 2019, the highest R&D intensities recorded by the pharmaceutical industry as a whole since at least 2000. Data are limited for earlier years, but among PhRMA member companies, annual R&D intensities averaged 18 percent from 1980 through 2010 and never exceeded 22 percent. 4 Since then, R&D intensity has increased among PhRMA firms just as it has for the industry as a whole, reaching 25 percent in 2017 before decreasing slightly in 2018. By comparison, average R&D intensity across all industries typically ranges between 2 percent and 3 percent. 5 R&D intensity in the software and semiconductor industries, which are generally comparable to the drug industry in their reliance on research and development, has remained below 18 percent (see Figure 1 ).
Average R&D Intensities for Publicly Traded U.S. Companies, by Industry

Pharmaceutical companies have devoted a growing share of their net revenues to R&D activities, averaging about 19 percent over the past two decades. By comparison, other research-intensive industries, like software and semiconductors, averaged about 15 percent.
Data source: Congressional Budget Office, using data from Bloomberg, limited to U.S. firms as identified by Aswath Damodaran, “Data: Breakdown” (accessed January 13, 2020), https://tinyurl.com/yd5hq4t6 . See www.cbo.gov/publication/57025#data .
R&D intensity is research and development spending as a share of net revenues (sales less expenses and rebates).
R&D = research and development; S&P = Standard and Poor’s.
There are several possible explanations for the increase in the industry’s R&D intensity over the past eight years. It could reflect the increased role of small drug companies, which have little revenue and, therefore, high ratios of R&D spending to net revenues. It could also indicate that the expected returns from investments in R&D have increased (if market conditions have changed) or that opportunities to develop new drugs have increased (if recent advances in science and technology have been particularly productive). Finally, it could reflect rising costs of R&D inputs, such as capital equipment and skilled labor. CBO has not evaluated the relative importance of those possibilities.
New Drug Development
Over the past decade, the pharmaceutical industry has introduced growing numbers of new drugs annually (see Figure 2 ). Between 2010 and 2019, 38 new drugs were approved each year, on average. That is about a 60 percent increase compared with the previous decade. Drug approvals reached a new peak in 2018, surpassing the record number of approvals of the late 1990s. (Counts of new drug approvals are a readily available but imperfect measure of output from the drug industry’s R&D spending. The measure does not reflect differences in the effectiveness of the new drugs relative to alternative treatments, or the number of people who might benefit from the new drugs.)
Average Annual Approvals of New Drugs by the FDA
Number of Drugs
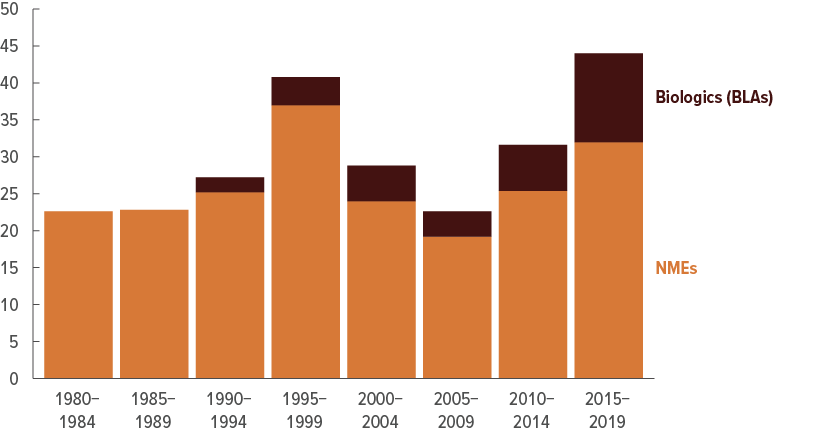
From 2015 to 2019, the FDA approved about twice as many new drugs as it did a decade earlier. Biologic drugs make up a growing share of FDA approvals.
Data source: Congressional Budget Office, using data from the FDA’s Center for Drug Evaluation and Research and the FDA’s Center for Biologics Evaluation and Research. See www.cbo.gov/publication/57025#data .
Until the 1990s, the FDA did not count biologics as a separate category; they were counted with NMEs.
BLA = biologic license application; FDA = Food and Drug Administration; NME = new molecular entity.
Information about the kinds of new drugs the pharmaceutical industry has introduced can be inferred from changes in retail spending across different therapeutic classes of drugs. When ranked by retail spending, therapeutic classes in which many expensive specialty drugs have been introduced over the past decade top the ranking, whereas classes in which the best-selling drugs are now available in generic form rank lower now than they did a decade ago. 6 Information about the kinds of new drugs the pharmaceutical industry may introduce in the future can be inferred from clinical trials under way.
Approval of New Drugs. Over the past five years, both R&D spending and drug approvals have increased substantially. The relationship between them is complex and variable (see Figure 3 ). Because it can take a decade or more of R&D spending to develop a new drug and successfully shepherd it through clinical trials, drug approvals lag behind the underlying R&D spending. That lag makes it difficult to interpret the relationship between R&D spending and new drug approvals. For instance, drug approvals declined over the 2000s despite steadily rising R&D spending over the preceding years, provoking concerns about a decline in the industry’s R&D productivity. Those concerns proved temporary, however. Despite flat R&D spending from 2008 through 2014, drug approvals began to increase around 2012.
R&D Spending and New Drug Approvals

Sustained increases in pharmaceutical R&D spending do not necessarily lead to rising numbers of new drugs. R&D spending also reflects rising costs of labor (skilled researchers) and capital (laboratory technologies).
Data source: Congressional Budget Office, using data from the FDA’s Center for Drug Evaluation and Research and PhRMA annual reports (various years). See www.cbo.gov/publication/57025#data .
Data for 1980–1983 are not shown because the five-year moving average cannot be calculated for the first four years of data.
FDA = Food and Drug Administration; NME = new molecular entity; PhRMA = Pharmaceutical Research Manufacturers of America; R&D = research and development.
a. A five-year moving average replaces the value for each year in an annual data series with an average over five consecutive years. (Here the arithmetic mean of each annual value and the preceding four is used.) A moving average is smoother than the underlying data series and is useful for reducing year-to-year changes unrelated to overall trends in the data.
That increase in drug approvals does not, by itself, indicate the extent to which the new drugs are particularly innovative (for instance, targeting illnesses in new ways) as opposed to improving only incrementally upon existing drugs. Furthermore, the recent trend of sharply rising R&D spending does not necessarily portend a continued high rate of drug introductions. A decline in clinical trials success rates, for example, could slow the rate of new drug introductions even while R&D spending continued to increase. Additionally, not all R&D spending is directed toward development of new drugs. Drug companies devote some R&D resources to finding effective new combinations of existing drugs, as with newer HIV treatments and preventatives, or to new drug-delivery mechanisms, such as insulin pumps.
Finally, the rise in the industry’s R&D spending does not provide information about the kinds of drugs that may be introduced in coming years. To some degree, that information can be inferred from descriptions of clinical trials currently in progress. But it cannot be known with any certainty which of those drugs will eventually make it to market.
Trends in Recent Drug Spending by Therapeutic Class. New or improved specialty drugs for diabetes, various cancers, autoimmune disorders (such as rheumatoid arthritis or multiple sclerosis), and HIV have propelled large retail-spending increases in the therapeutic classes for those illnesses (see Figure 4 ). Many of the new specialty drugs are biologics, based on living cell lines rather than chemical active ingredients. For HIV, the new antiretroviral therapies have been combinations of specialty drugs that simplify treatment.
Total U.S. Retail Drug Spending by Therapeutic Class, 2009 and 2019
Billions of 2019 dollars
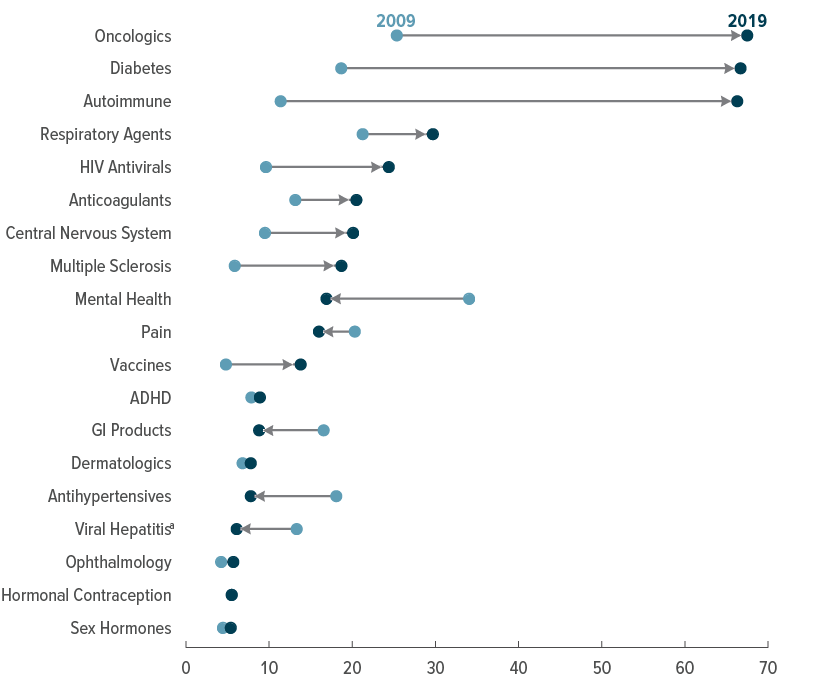
New drugs can lead to large increases in retail spending because they have higher prices, they are in high demand, or both. Spending decreases can result when patent protection expires on leading drugs and low-cost generic versions are introduced.
Data source: Congressional Budget Office, using data from IQVIA Institute for Human Data Science, Medicine Spending and Affordability in the United States: Understanding Patients’ Costs for Medicines (August 2020), Exhibit 24, https://tinyurl.com/5655tnoc ; IMS Institute for Healthcare Informatics, Medicines Use and Spending Shifts: A Review of the Use of Medicines in the U.S. in 2014 (April 2015), p. 40, https://tinyurl.com/3bk9oufn , and Medicine Use and Shifting Costs of Healthcare: A Review of the Use of Medicines in the United States in 2013 (April 2014), Appendix 8, https://go.usa.gov/xsaFR . See www.cbo.gov/publication/57025#data .
Therapeutic classes in the figure are ranked in order of 2019 spending. The figure excludes “other cardiovasculars” (ranked 12th in 2019, with total spending of $10.1 billion) because 2009 data for that class could not be found.
Retail spending overstates actual spending and revenues received by manufacturers, because it does not include rebates paid by those manufacturers.
ADHD = attention deficit hyperactivity disorder; GI = gastrointestinal.
a. Viral hepatitis entered the list of the top 20 therapeutic classes by retail spending in 2014; therefore, spending levels for that year have been substituted for 2009 levels.
Some of the therapeutic classes that have experienced large spending increases feature new drugs with relatively large populations of patients or new treatments for chronic conditions that can be therapeutically managed but require continued treatment. (As a result, drugs for chronic conditions typically sell in steady quantities.) Other such classes include new drugs with relatively small numbers of potential patients or shorter treatment durations but that have high prices per unit of treatment. High prices may reflect demand that is relatively insensitive to price because of the serious nature of the illness and coverage of those drugs by insurance plans. For example, prices for oncology drugs tend to be high.
In some cases, observed increases in retail spending overstate increases in net revenues to the manufacturer because they do not account for unobserved rebates. 7 Rebates tend to be higher for drugs for which several competing therapies are available. (Larger rebates correspond with lower net prices.) Thus, rebates on diabetes drugs tend to be considerably higher—as a percentage of the retail price—than they do for oncology drugs, which are not highly substitutable.
Several therapeutic classes that contain top-selling drugs developed in the 1990s experienced decreases in retail spending from 2009 to 2019 as they faced competition from generic versions. Those blockbuster small-molecule drugs include atypical antipsychotics, ACE inhibitors, and proton pump inhibitors. The therapeutic classes containing those drugs—mental health, antihypertensives, and gastrointestinal products, respectively—experienced large declines in retail spending. One therapeutic class, lipid regulators (the class that includes statins), experienced such a decrease that it no longer appears among the top 20, ranked by retail spending. Those declines reflect widespread use of the new generic versions of those drugs.
One therapeutic class has experienced a decline in retail spending for a different reason. Viral hepatitis only entered the top 20 in 2014, coinciding with the introduction of several highly effective—and high-priced—new treatments for hepatitis C. In contrast to the spending declines described above, the decline in retail spending on viral hepatitis drugs is attributable to a combination of factors. First, newer, lower-priced drugs have since been introduced, lowering the average price in that class as they have gained market share. Second, the number of prescriptions has declined: As the treatments have been administered, the number of potential patients has fallen. That is because the new drugs successfully treat about 95 percent of patients with chronic hepatitis C infection. 8 By contrast, older, less expensive therapies were successful in far fewer patients and had severe side effects in many cases.
Types of New Drugs in Development. Information about the kinds of drugs that may be approved in coming years can be gleaned from data on recent clinical trials. That information suggests that drug companies are emphasizing treatments for cancer and nervous system disorders like Alzheimer’s disease and Parkinson’s disease. Among human clinical trials in progress as of 2018, drugs in those two therapeutic classes accounted for more than twice as many trials as did drugs in the next three classes combined (vaccines; pain, including arthritis therapies; and dermatologics.) 9
The 2020–2021 coronavirus pandemic has spurred the development of vaccines to halt the spread of COVID-19, the disease caused by the coronavirus. In addition to R&D spending by the private sector, the federal government has provided support to the private sector to develop vaccines to address the pandemic (see Box 2 ).
Federal Funding to Support the Development of a COVID-19 Vaccine
The federal government can directly support private vaccine development in two primary ways, either by covering the costs of research and development (R&D), or by committing in advance to purchasing a successful vaccine contingent upon a firm achieving specified development goals. Under the first method, the government would supply R&D funding that would ordinarily come from the pharmaceutical firms themselves, from venture capital investments, or from other sources outside the firm. That method might be better suited to cases in which the R&D effort had a relatively high risk of failure and an expected return that would be too low to attract private investment. The rationale for government funding in such cases would depend on whether the expected value to society—rather than to private investors—exceeded the cost of the funding. However, a drawback of such funding is that the outside funder—including the government, in this case—cannot observe the innovator’s private costs and may pay more than necessary for developing the vaccine.
Under the second method—that is, agreeing to a future purchase of a specified number of vaccine doses at a specific price—the government would become the source of demand that ordinarily comes from the market. Such an advance-purchase agreement might be preferable in cases in which the government planned to purchase the new product in large quantities regardless of the amount of financial support it provided for R&D. It might also be preferable in cases in which a variety of approaches to developing the product are available, but with much uncertainty about which approach is best. An advance-purchase agreement would also ensure the developer a certain amount of revenues in cases in which the government was supporting the development of multiple, competing products simultaneously. By offering advance purchase contracts to vaccine manufacturers—the promise of future payment conditional on a successful vaccine being developed—the government can provide greater certainty to pharmaceutical firms undertaking risky investments in R&D for vaccines.
In May 2020, the Department of Health and Human Services initiated “Operation Warp Speed,” a collaborative effort involving the Centers for Disease Control and Prevention, the Food and Drug Administration (FDA), the National Institutes of Health, and the Department of Defense, with funding provided through the Biomedical Advanced Research and Development Authority (BARDA). Through Operation Warp Speed, the federal government has provided more than $19 billion in assistance to seven private pharmaceutical manufacturers to develop and produce a vaccine or treatment for COVID-19, the disease caused by the coronavirus (see the table below). 1 As of March 2, 2021, five of those seven companies accepted up-front funding for research and clinical trials. Five of the seven companies accepted advance funding aimed at helping manufacturers ramp up their production capabilities while their potential vaccines were still in development; a sixth accepted funding to develop the capacity to manufacture another firm’s vaccine after it received emergency use authorization. Finally, six of the seven manufacturers signed advance-purchase agreements. Two of the companies with vaccines that have received emergency use authorizations have received additional funding for selling more doses than were guaranteed by advance-purchase agreements.
The parallel execution of several stages of development that would usually be conducted in sequence, such as combining phase I and phase II clinical trials or building manufacturing capacity while the trials are still under way, has allowed pharmaceutical manufacturers to advance much more quickly through the development process than is typical for vaccines. 2 One year after the first case of COVID-19 was diagnosed in the United States, three of the vaccines supported by BARDA funding had received emergency use authorizations from the FDA, and two other vaccines were in phase III clinical trials. (It ordinarily takes several years of research and testing before a candidate vaccine enters phase III clinical trials. 3 Seasonal influenza vaccines take much less time to develop and approve because their technologies, and the regulatory and licensing procedures for those vaccines, have been used before.) According to the World Health Organization, more than 200 candidate COVID-19 vaccines were in development in February 2021. 4
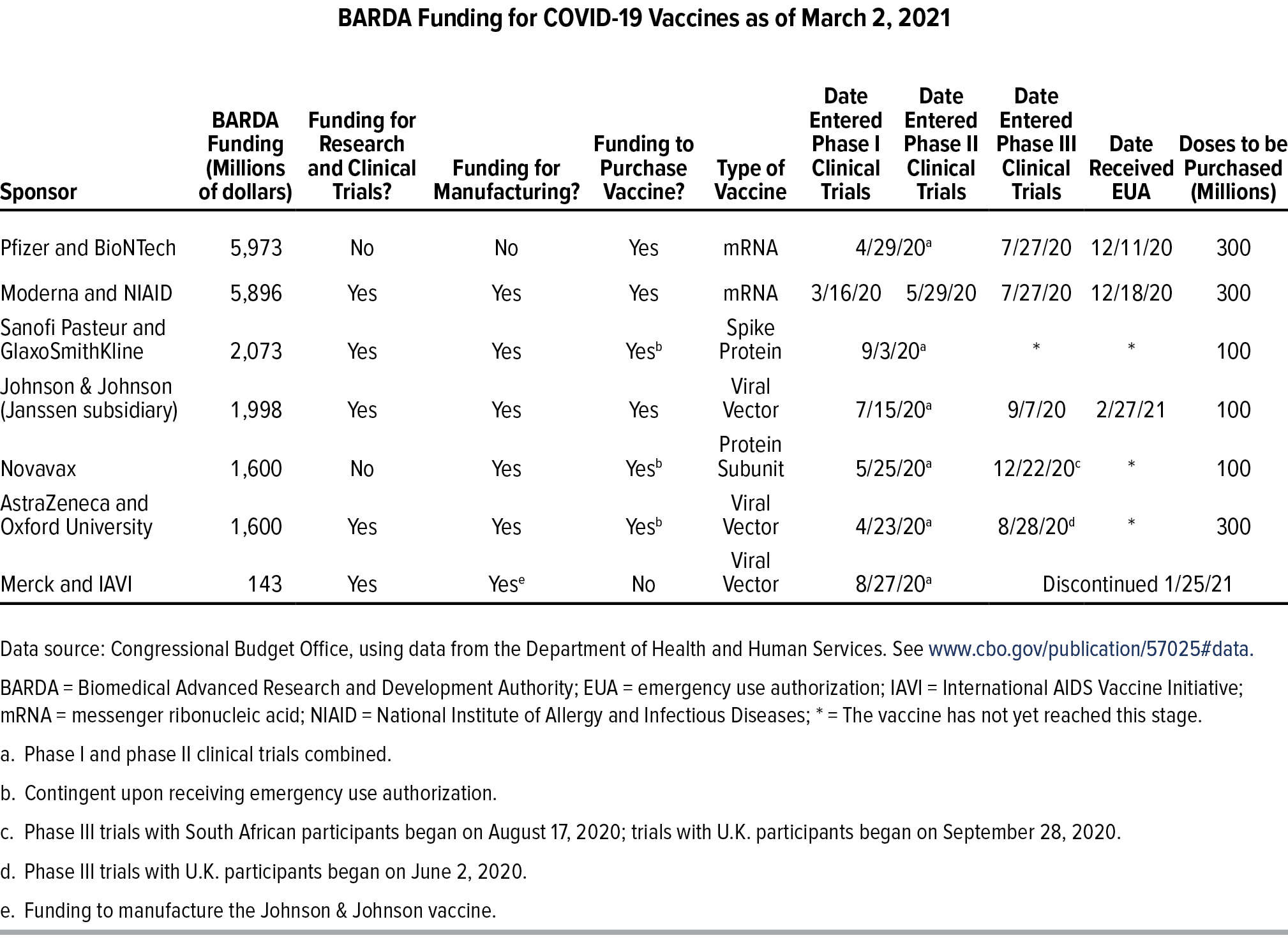
1 . Most of the manufacturers have also received research support from or signed advance-purchase agreements with the European Union, several national governments, and two global partnerships supported by foundations and other donors (Coalition for Epidemic Preparedness Innovations and Gavi, the Vaccine Alliance). See, for example, Christopher M. Snyder and others, “Designing Pull Funding for a COVID-19 Vaccine,” Health Affairs , vol. 39, no. 9 (September 2020), pp. 1633–1642, https://doi.org/10.1377/hlthaff.2020.00646 .
2 . See Nicole Lurie and others, “Developing Covid-19 Vaccines at Pandemic Speed,” New England Journal of Medicine, vol. 382 (May 21, 2020), pp. 1969-1973, https://doi.org/10.1056/NEJMp2005630 .
3 . See Wellcome Trust, “The 5 Stages of Vaccine Development” (accessed January 15, 2021), https://tinyurl.com/y6rxbbuf .
4 . See World Health Organization, “COVID-19 Vaccines” (accessed March 24, 2021), https://tinyurl.com/fpdcc777 .
Factors That Influence R&D Spending
Pharmaceutical companies invest in R&D in anticipation of future profits. For each drug that a company considers pursuing, anticipated returns depend on three main factors: the expected lifetime global revenue from the drug (minus its manufacturing and marketing costs), the new drug’s likely R&D costs, and policies that affect the supply of and demand for prescription drugs. When the anticipation of future profits is higher, companies invest more in R&D and produce more new drugs, CBO estimates. Similarly, if expectations about prices and profits were lower, companies would invest in less R&D, and fewer drugs would be developed (see Box 3 ).
Effects of Changes in Expected Profitability on the Introduction of New Drugs
If expected profitability of new drugs declined—because of a change in federal policy, a shift in demand or supply, a revision in the balance of power between drug companies and drug buyers, or for any other reason—the expected returns on drug R&D would decline as well. Lower expected returns would probably mean fewer new drugs, because there would be less incentive for companies to spend on R&D. (If expected profitability were to rise, the opposite effects would occur.) Expectations about returns on R&D partly depend on expectations of prices that future drugs could command—which, in turn, partly depend on current drug prices and influences on those prices.
The Congressional Budget Office’s analysis of H.R. 3 in the 116th Congress illustrates those effects. That bill would have required the Secretary of Health and Human Services to negotiate prices for drugs—primarily those for which spending was highest—and to subject manufacturers who did not participate in negotiations to an excise tax. In that analysis, CBO concluded that the bill would reduce drug companies’ expectations about future revenues because of the new negotiating leverage of the Secretary. The prospect of such lower revenues would make investments in R&D less attractive to pharmaceutical companies. CBO estimated that under the bill, approximately 8 fewer drugs would be introduced to the U.S. market over the 2020–2029 period and about 30 fewer drugs over the subsequent 10 years. 1 Those estimates were in the middle of the distribution of possible outcomes, in CBO’s assessment, and were uncertain. CBO’s analysis is in line with a broader literature that has found a positive relationship between drug prices and R&D efforts. 2
1 . See Congressional Budget Office, letter to the Honorable Frank Pallone Jr. regarding the budgetary effects of H.R. 3, the Elijah Cummings Lower Drug Costs Now Act (December 10, 2019), www.cbo.gov/publication/55936 .
2 . See Margaret E. Blume-Kohout and Neeraj Sood, “Market Size and Innovation: Effects of Medicare Part D on Pharmaceutical Research and Development,” Journal of Public Economics, vol. 97 (January 2013), pp. 327–336, https://doi.org/10.1016/j.jpubeco.2012.10.003 ; Daron Acemoglu and Joshua Linn, “Market Size in Innovation: Theory and Evidence From the Pharmaceutical Industry,” Quarterly Journal of Economics, vol. 119, no. 3 (August 2004), pp. 1049–1090, https://doi.org/10.1162/0033553041502144 ; and Pierre Dubois and others, “Market Size and Pharmaceutical Innovation,” RAND Journal of Economics, vol. 46, no. 4 (Winter 2015), pp. 844–871, https://doi.org/10.1111/1756-2171.12113 .
Anticipated Revenues
A company’s expectations about the revenues it could earn from a drug depend on the prices that the company anticipates the drug could command in various markets around the world and the quantities that the company anticipates might be purchased at those prices. Those expectations are informed by the prices and sales volumes observed for existing drugs in various markets. For established drug companies, current revenue streams from existing products also provide an important source of financing for their R&D projects.
How Revenue Expectations are Formulated. A company develops its expectations about a potential drug’s lifetime future revenues based on the drug’s potential market size, which depends on the prices it might command in sales to different patient groups and in negotiations with payers, domestically and abroad. In that sense, the prices of existing drugs—including variations in prices to different patient populations—help determine R&D spending on future drugs. (The converse is not true: In CBO’s assessment, current R&D spending does not influence the future prices of the drugs that result from that spending.)
Revenues generated by existing drugs provide information about the potential market size for new drugs by indicating consumers’ and insurance plans’ willingness to pay for drug treatments. The number of prescriptions for those drugs support inferences about the number of potential patients, their propensity to use drug therapies at the observed prices, and the popularity of competing therapies.
Sales revenues from other unrelated drugs also help companies form expectations about market size. They reveal information about the magnitude of drug-treatment costs that the market currently tolerates, both in general and for various conditions that will have more or less in common—with regard to duration, severity, or effects on quality or length of life—with the conditions the new drug would treat.
Expected revenues also depend on anticipated unit sales in different markets around the world. Those quantities are determined by the number of potential patients for the drug in those markets, the shares of those populations that might buy the drug at the prices the manufacturer envisions for those markets (taking into account any substitute drugs that might be available), and the number of prescriptions a course of treatment would require.
Once a new drug has been approved, CBO expects that its developer would set its price in a forward-looking fashion, meaning the price is set to maximize the net revenues from the drug without regard to how much it cost to develop.
Real (inflation-adjusted) pharmaceutical revenues increased sharply from the mid-1990s until around the mid-2000s, when patents on a number of blockbuster drugs expired and lower-cost generic equivalents were introduced. Revenues then declined slightly from the mid-2000s through the mid-2010s, a result of those patent expirations and the 2007–2009 recession. Revenue growth returned with the introduction of some expensive new drugs (see Figure 5 ).
Worldwide and Domestic Revenues of PhRMA Member Firms
Billions of 2019 Dollars
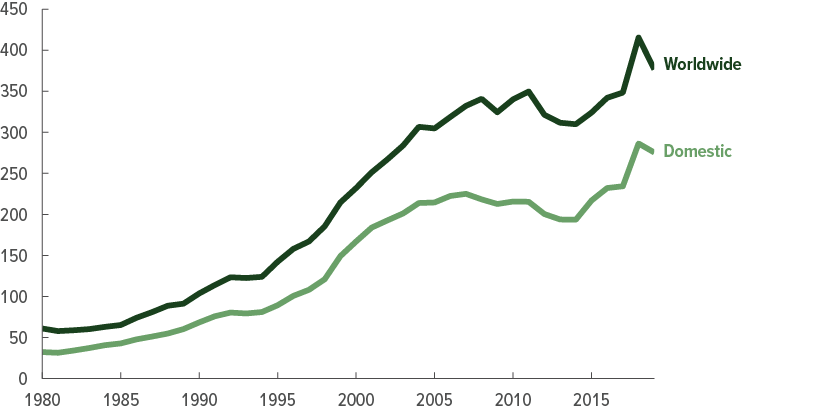
Revenues from drug sales have grown substantially since 1980, although that growth was interrupted by patent expirations of some widely used drugs and by the 2007–2009 recession. Revenue growth has since resumed, in part due to expensive new drugs.
Data source: Congressional Budget Office, using data from PhRMA, 2019 PhRMA Annual Membership Survey , Table 4 (PhRMA, 2019), https://tinyurl.com/ycvneve7 (PDF, 2.15 MB). See www.cbo.gov/publication/57025#data .
PhRMA revenue data reflect payments received by manufacturers, excluding cash discounts, Medicaid rebates, returns, and allowances for marketing expenses.
PhRMA = Pharmaceutical Research and Manufacturers of America.
Revenues as Source of Funding for R&D. In the pharmaceutical industry, revenues have traditionally been an important source of R&D financing for established companies with brand-name drugs to sell. Brand-name drugs can generate large volumes of cash because their manufacturing and distribution costs are typically very low relative to their sales revenues. Established companies appear to prefer to finance their R&D with current revenues whenever possible rather than to rely on outside funding sources such as venture capital. 10 Outside financing involves transactions costs as well as other implicit costs, such as compensation for risks borne by outside investors who cannot perfectly monitor a firm’s efforts and skills. 11
The share of R&D funded directly by revenues has declined in recent years because an increasing amount of R&D is now conducted by research-oriented drug companies with few or no products on the market. Over the past decade, small or emerging drug companies have developed a rising share of new drugs. Those companies have relatively little revenue (some have none at all), and most of them must seek outside financing, such as venture capital, and collaborative agreements with larger drug companies. Although venture capital still only finances a small share of the drug industry’s R&D spending in total, it supports a much larger share of the R&D spending of smaller firms than of large established companies.
Drug development also occurs in university research labs. In addition to grants funded by the National Institutes of Health (NIH) that many universities receive for performing basic biomedical research, universities may collaborate with (and be funded by) private drug companies to perform applied research toward the development of new drugs. 12 The funding for that R&D may come predominantly from revenues, as the collaborations typically involve established pharmaceutical companies. 13
R&D Costs of a New Drug
R&D spending is also influenced by the expected costs of developing a new drug, including those incurred in the preclinical research phase and in clinical trials. In addition to those out-of-pocket expenses, drug companies incur capital costs that result from tying up funds in the drug-development process for years before they generate earnings from those investments. Those capital costs reflect the returns that the funds could have earned if they had been invested in other ways.
Development of a drug that will eventually reach the market often entails a decade or more of R&D expenditures. Each successive phase of clinical trials requires increasing amounts of spending. Drug developers can reassess their commitment at each stage, and a drug’s expected value may change as more is learned in clinical trials or as market conditions change—that is, there is an option value to continuing. Companies will not necessarily cancel a drug project even if its likely future costs exceed its likely value when that assessment is made, because the expected value might rise with additional information about the drug or its market.
Pharmaceutical research is inherently risky and canceled or failed projects are a normal part of any drug development program. Companies initiate drug projects knowing that most of them will not yield a marketable drug. Some drugs developed in the preclinical phase never enter clinical trials, and of those that do, only about 12 percent reach the market (recent estimates range from 10 percent to 14 percent). 14
Estimates, from multiple sources, of average R&D expenditures per new drug range from less than $1 billion to more than $2 billion. Those estimates all include capital costs as well as expenditures on drugs that did not make it to market. The different estimates are averages over different samples of companies and drugs—that is, they depend on analytical and sampling choices made by the researchers producing those estimates and are best interpreted as illustrative of the general conclusion that developing new drugs is expensive and subject to high rates of failure.
Preclinical Phase. Although drugs spend much less time in preclinical development than they do in clinical trials, a company’s total preclinical R&D expenditures typically constitute a considerable share of its total R&D spending. That is because companies typically develop many potential drugs in the preclinical phase that never enter or complete clinical trials. According to one estimate using data provided by large pharmaceutical firms, preclinical development accounted for an average of 31 percent of a company’s total expenditures on drug R&D, or $474 million per approved new drug. 15
When capital costs were taken into account, the share of R&D spending in the preclinical phase rose to 43 percent. Any return on R&D spending on early, preclinical drug development must await successful completion of both the preclinical phase and the clinical trials that follow. As a result, the lag between investment and return is longer for R&D spending that occurs in the preclinical phase than for spending in clinical trials. (For drugs that do not reach the market, no return is realized, although lessons learned from those efforts may aid the development of other drugs.) According to one study, the preclinical phase takes an average of about 31 months, followed by around 95 months, on average, for clinical trials—or about 10.5 years from start to finish. 16 Other estimates differ; in a sample of 10 cancer drugs, for example, one study found that the median time from discovery to approval was 7.3 years. 17 Those numbers are measures of central tendency: Some drugs are brought to market in less time. 18
Clinical-Trials Phase. The costs to conduct clinical trials on a drug are higher than those to conduct the preclinical phase because trials involve the contributions of many more people for a longer time. Clinical trials occur in several phases:
- Phase I trials (also known as human-safety trials) test a potential new drug at different dosage levels, generally in a small group of healthy volunteers in order to assess its safety in humans. For drugs with high levels of expected toxicity, phase I trial subjects are people with the targeted illness.
- Phase II trials are larger and include only people with the medical condition the drug is intended to treat. Phase II trials assess the drug’s biological activity and identify and characterize any side effects.
- Phase III trials are larger still and assess a drug’s clinical effectiveness. They can take years to complete. The smaller a drug’s expected therapeutic effect relative to a placebo, the larger the number of patients that are needed in the drug’s phase III trials so that the drug’s true effect (if any) can be distinguished from random variation in patient outcomes.
- Phase IV trials (also known as pharmacovigilance trials) may be conducted after a new drug has reached the market. They look for side effects not seen in earlier trials and measure a drug’s efficacy over longer periods of use than were studied in earlier trials.
Generally, only drugs that have successfully navigated the first three phases can be considered for FDA approval, although regulators sometimes approve new drugs without a phase III trial. (Of the 59 drugs approved in 2018, 7 did not undergo phase III trials before approval.) 19 In some cases the FDA may require a phase IV trial after the drug is approved to detect adverse reactions that might not be observed until a drug is in wider use. Drug companies also might choose to conduct phase IV trials to show (for marketing purposes) the superiority of their product over other available drug therapies.
Few of the drugs that enter clinical trials are ultimately approved; some fail in clinical trials, and others are set aside when a company decides to focus on more promising drugs. In a few cases, drugs submitted for approval are rejected by the FDA. In one sample of drugs in clinical trials, researchers found that for every 100 drugs entering phase I trials, around 60 advanced to phase II trials, just over 20 entered phase III trials, and only about 12 gained FDA approval. 20 Such winnowing is reflected in the average R&D cost per approved drug, which includes all of the R&D spending on drugs that do not reach the market.
Costs tend to rise in each successive phase of development. In the sample just described, companies spent an average of about $1,065 million in clinical trials per approved new drug (more than twice the amount spent in the preclinical research phase). Spending averaged $28 million in phase I, $65 million in phase II, and $282 million in phase III. 21 For each drug that completed the first three phases of clinical trials, the average total cost of those trials was about $375 million. The remaining $690 million (of the $1,065 million in average total spending on clinical trials) reflects companies’ contemporaneous spending on drugs that failed in clinical trials or were otherwise set aside.
Capital Costs of R&D. In addition to the cost of preclinical research and clinical trials, drug companies incur costs by forgoing other opportunities for investment with money spent on clinical trials. Because drug companies’ R&D spending on a drug occurs over many years, those capital costs are substantial and can approach the value of actual R&D expenditures to develop a new drug.
Estimates of Total R&D Costs. Three recent studies have estimated the average R&D cost per new drug. They all measure R&D costs the same way: They add up all of the R&D spending by each company in their sample—not only its spending on the sampled new drug but the company’s spending on other drugs that were being developed at the same time but that did not reach the market. The studies also all apply a cost-of-capital adjustment to each company’s R&D spending to reflect the lag between investment and return on investment. 22 Despite their methodological similarities, the studies’ estimates range from $0.8 billion to $2.3 billion of R&D spending per new drug.
Differences in sample selection and data sources appear to be important sources of variation in those estimates. The largest estimate, $2.3 billion (from a 2016 study, expressed here in 2019 dollars), includes around $900 million in preclinical research spending and $1.4 billion for clinical trials. 23 Those estimates are based on a sample of 106 randomly selected drugs from 10 large pharmaceutical firms, 5 of which are ranked among the industry’s top 10 by sales revenues, with an additional 3 ranked in the top 50 but outside the top 25. 24 That widely cited study is the latest in a series of similar studies the authors have published over the past three decades. Because the R&D expenditures reported by the sampled firms are not publicly available, it is difficult to evaluate the extent to which the results of those studies are affected by the selection of the sample and other aspects of the method of collecting data. 25 An independent effort to replicate an earlier iteration of the study found similar results, however. 26
The second study, which was conducted in part to provide an alternative to those 2016 estimates, found an average R&D cost of $1.2 billion (expressed here in 2019 dollars), with expenditures for individual drugs ranging from $137 million to $5.8 billion. 27 That upper bound, based on one outlier drug accounting for $2.2 billion in actual R&D outlays and $3.6 billion in capital costs, skews the average estimate upward. The median R&D cost, unaffected by the outlier, is $0.9 billion.
The sample in that study consisted of 63 drugs (developed by 47 different companies) out of the 355 drugs that the FDA approved between 2009 and 2018. R&D expenditure data for those 63 drugs are publicly available (unlike the data used in the 2016 study). The sample skews toward smaller firms—although the same is now true of drug development generally—and the authors caution that their sample may overrepresent drugs approved between 2014 and 2018 and those in certain therapeutic areas, first-in-class drugs, orphan drugs, and therapeutic agents that received accelerated approval. The R&D data include the companies’ spending on drugs that did not reach the market.
In the third study, researchers limited their sample to new cancer drugs from companies with no previously approved products. They found an average cost of $0.9 billion per approved drug (expressed here in 2019 dollars). 28 Notably, that study excluded R&D spending by firms that had not developed any approved drugs, and thus the study underestimates R&D spending on failed drugs and, by extension, expected costs per new drug. Median observed R&D costs in that sample were about $0.8 billion per new drug, with estimates for individual drugs ranging from about $212 million to $2.7 billion including capital costs. Those estimates include the developers’ total R&D spending while the approved drugs were under development, including that on failed drugs.
Trends in R&D Costs . R&D costs have increased by about 8.5 percent per year over roughly the past decade. 29 The increase in average R&D costs might reflect changes in the kinds of drugs being developed or in the number of drugs in costly clinical trials. If success rates for new biologic drugs were lower than for traditional, small-molecule drugs, or if R&D spending on failed drugs was higher for biologics, that would also contribute to higher average R&D costs.
Some evidence suggests that average success rates may indeed have declined. The 2016 study found that fewer than 12 percent of the drugs entering phase I clinical trials ultimately reached the market, but it reported success rates in excess of 20 percent for drugs developed in the 1980s and 1990s. 30 However, other evidence suggests that the overall success rate of clinical trials has not declined. 31
Another possible factor in rising R&D costs is that it has become harder to recruit candidate patients into some kinds of clinical trials. 32 For example, prospective patients might be less interested in taking a chance on untested treatments in clinical trials when approved treatment options are relatively effective already. And, in some therapeutic classes, it has become more difficult to demonstrate that a new drug would improve on the existing standard of care. For example, advances in oncology treatments have extended cancer patients’ expected lifespans. As a result, clinical trials on potential cancer drugs have had to be expanded or extended so that the treatment effect on the lifespans of patients can be estimated with suitable precision. That is, because oncology treatments have become more effective, it now takes longer, on average, to observe a given number of deaths in a clinical trial. 33
Public Policy
Federal policy influences pharmaceutical companies’ R&D spending, both in magnitude and direction. (Policies in other countries and at other levels of government can also affect such spending. Those policies are outside the scope of this report.)
Policies around federal health care programs and subsidies most directly affect the demand for new drugs. Other policies affect the supply of new drugs (federal support for basic research, tax treatment of R&D spending, and those policies that affect market exclusivity). Still other areas of federal policymaking affect both supply and demand (vaccine policies and regulatory policies).
Changes in policy that increased the demand for pharmaceuticals or encouraged their supply would tend to make R&D activity a more attractive investment. Policy changes in the opposite direction could make it a less appealing one.
Federal Health Care Programs and Subsidies . A variety of federal health care programs and subsidies increase demand for health care services and products, including prescription drugs. Such initiatives indirectly stimulate spending on drug R&D. In particular, the federal government—through Medicare, Medicaid, TRICARE, the Veterans Health Administration, the Children’s Health Insurance Program, and health insurance marketplaces established by the Affordable Care Act—purchases or subsidizes the purchase of a substantial number of prescription drugs on behalf of retirees, veterans, persons with disabilities, and low-income households. Taken together, federal and state expenditures on prescription drugs accounted for about 40 percent of total U.S. retail expenditures on prescription drugs in 2019. 34
Changes to those programs would influence R&D spending. For instance, when Medicare Part D (Medicare’s prescription drug benefit) was implemented in 2006, sales of prescription drugs to enrollees increased considerably. In addition, for Medicare enrollees with full Medicaid benefits, coverage of prescription drugs shifted from Medicaid to Medicare Part D, increasing the average prices paid for those enrollees’ brand-name drugs. Those increases in current and anticipated revenues encouraged the industry to develop new drugs for the Medicare population. Between 2003 and 2010, the number of drugs entering phase I clinical trials increased by roughly 50 percent in therapeutic classes with relatively high sales to Medicare enrollees. That increased development activity eventually led to increases in the number of drugs in those classes. 35
The federal government also increases demand for prescription drugs by subsidizing employment-based health insurance: An employer’s contribution toward the cost of that coverage is excluded from an employee’s taxable income, effectively reducing its price to the employee. As a result, many people select more generous health insurance coverage than they otherwise would, which increases their spending on health care (including prescription drugs) and indirectly stimulates pharmaceutical R&D. That stimulus would disappear if the tax subsidy on employment-based health insurance was eliminated. The size of the effect that would have on R&D spending would depend on how the elimination of the subsidy would affect individuals’ choices of health insurance coverage. 36
Support for Basic Research. The federal government is the primary funder of basic research in biomedical sciences. That research ultimately increases the supply of new drugs because drug companies rely on the findings from that research—for example, the identification of disease targets toward which new drug therapies can be aimed. That basic research creates knowledge that, in effect, reduces private companies’ R&D costs and stimulates private investment in R&D, because it expands the set of potentially profitable drug development opportunities. In particular, increases in basic health-related research at the NIH or other federal research agencies have been found to increase private drug R&D in therapeutic classes related to that basic research. 37
The rationale for public investment in basic biomedical research is that private firms’ incentives to invest in it are muted. Basic research generates knowledge (such as the identification of a disease target) that is not readily embodied in a marketable product (such as a drug). The more of that information a company could keep to itself, the greater its value to the company—and the stronger the company’s incentive would be to invest in that research. But because information can be communicated at low cost, it can be difficult to contain within a firm. Private companies tend to be reluctant to conduct basic research such as identifying a new disease target, because it would be difficult to keep much of the value of that discovery for themselves. For example, once a disease target is known, multiple companies (not just the company that identified it) might be able to develop drugs aimed at that target. That weakens private incentives to invest in basic research and, as a result, private firms do too little of it from the perspective of society as a whole (meaning that the social benefit if they performed additional basic research would be greater than the cost).
The Role of NIH-Funded Research. In the past two decades, federal funding for NIH has totaled over $700 billion. 38 Much of that funding has supported basic research (in genomics, molecular biology, and other life sciences) that has identified new disease mechanisms. Federal support for NIH nearly doubled between 1995 and 2003, rising from $18 billion to about $37 billion (see Figure 6 ). Federal funding for NIH declined (in inflation-adjusted dollars) each year from 2003 to 2015, when that funding was about $33 billion. With real annual increases over the subsequent five years, funding for NIH reached $41 billion in 2020.
Federal Funding for the National Institutes of Health, Fiscal Years 1995 to 2020

Large increases in funding for NIH—the locus of much of the federal government’s basic biomedical research support—in the late 1990s and early 2000s preceded a decade of declining funding. Since 2016, NIH funding has increased annually.
Data source: Congressional Budget Office, using data from National Institutes of Health, Office of Budget. See www.cbo.gov/publication/57025#data .
NIH = National Institutes of Health.
Between 2010 and 2016, every drug approved by the FDA was in some way based on biomedical research funded by NIH. 39 In many cases, new drugs targeted a disease mechanism that had been identified by advances in basic science resulting from that funding. Indeed, most of the important new drugs introduced by the pharmaceutical industry over the past 60 years were developed with the aid of research conducted in the public sector. 40 Publicly funded basic science thus provided the foundation upon which complementary work on the applied science of drug development could be undertaken by the private sector.
How NIH-Funded Research Affects Private R&D. Empirical studies find that public-sector research tends to increase private R&D rather than to decrease it—that is, they are complements, not substitutes. 41 Several recent studies have associated increases in NIH-funded basic research with increased private R&D efforts. 42 One study found that in the decade following an increase in NIH funding, private R&D spending grew by about eight times as much as the increase in that funding. 43 Another study found that for every two NIH research grants, about one new private-sector patent was awarded. 44
The complementary relationship between public and private R&D spending arises mainly because NIH funding focuses on basic research that leads to the discovery of new drugs, whereas private spending focuses on applications of such research. Private R&D spending on clinical testing, incremental innovation, product differentiation, and safety all follows from basic research.
That relationship is complicated by two factors. First, the distinction between basic and applied research is not well defined, and the likelihood that federal research spending crowds out private R&D spending varies by type of research. The risk of crowding out is greater when the government funds research whose potential commercial applications are obvious and valuable, as was the case when federal and private research labs raced to map the human genome. Second, federal research spending can also indirectly crowd out private spending by increasing the demand for skilled researchers. That could cause an increase in research labor costs in the private sector as well as in the public sector. 45
Tax Treatment of R&D Spending. The tax code increases the supply of new drugs in two ways: First, it provides tax credits for certain R&D expenditures (including credits available to all types of companies and credits specifically for developing drug treatments for uncommon diseases). Second, it allows all types of companies to deduct expenditures that are not eligible for the credits as business expenses in the year they are made. Both incentives encourage R&D spending by reducing its cost to the company.
Tax Incentives. The research and experimentation tax credit, available to all types of companies for certain qualifying R&D expenditures, directly reduces the amount of income tax a company owes. 46 That tax credit has been modified over time and was made permanent by the Consolidated Appropriations Act, 2016 (Public Law 114-113). 47 Some of the increase in R&D spending by pharmaceutical industries over the past several decades might have been a response to changes in that credit. In addition, the Orphan Drug Act (P.L. 97-414), enacted in 1983, created a tax credit to encourage the development of drugs to treat relatively uncommon diseases. Companies can also choose to deduct the cost of R&D investments immediately rather than over the life of the investment. Many companies use both tax credits and the ability to accelerate their deductions for investments in R&D, although only one tax preference may be used for any particular investment expense.
Effects of the 2017 Tax Act. The net effect of P.L. 115-97 (originally called the Tax Cuts and Jobs Act and called the 2017 tax act in this report) on R&D investment is uncertain. Investment in R&D is encouraged by the reduction in the top corporate tax rate from 35 percent to 21 percent because earnings on new drugs would be taxed at a lower rate.
Investment is discouraged by changes in how deductions for R&D expenditures can be taken. The act is expected to reduce the value of tax deductions for R&D when they take effect. Beginning in 2022, companies will deduct their annual R&D costs over a five-year period rather than receiving the full tax deduction in the year the expenses are incurred. That discourages investment in R&D because the value of that deduction will decline. The reduction in the top corporate tax rate will further reduce the value of the tax deduction.
The 2017 tax act also reduced the tax credit created by the Orphan Drug Act from 50 percent to 25 percent of the cost of clinical trials. 48 When combined with the lower tax rate, that change will reduce the first-year tax benefits for R&D spending on orphan drugs by about 40 percent. (Costs applied to the tax credit for orphan drugs cannot also be applied to the research and experimentation credit, nor can they be deducted as expenses.) That change will also discourage investment in drug R&D.
Policies Affecting Market Exclusivity. The federal government has adopted a variety of policies that grant periods of market exclusivity to manufacturers in order to increase the supply of new drugs. During those periods, the average prices for those new drugs are higher than they will be later, once lower-priced, generic versions are allowed to enter the market. The return on R&D spending provided by those higher prices encourages companies to develop new drugs. That incentive is not unlimited: A manufacturer only receives market exclusivity over its own drug. There may be competing drugs in the same therapeutic market, and companies may introduce other new drugs into that market, providing they do not infringe the existing drugs’ patents.
The primary way that the federal government grants innovators temporary market exclusivity is through the U.S. patent system. Most patents expire 20 years after the date on which the patent application was filed, but pharmaceutical companies can receive several additional years of patent protection in recognition that patented drugs cannot be sold until they complete clinical trials. (Drug patent applications are often filed before the drug enters clinical trials, because disclosures from those trials could be considered “prior art” that might invalidate a patent if its application were filed after those disclosures occurred.) In recognition that a drug might spend several years of its market exclusivity in clinical trials, earning no revenue, the Hatch-Waxman Act (P.L. 98-417) allows pharmaceutical companies to seek up to five years of additional patent protection.
Pharmaceutical companies can also receive additional exclusivity—distinct from that afforded by patents—for drugs that treat relatively uncommon diseases. The Orphan Drug Act, enacted in 1983, offers seven years of market exclusivity (for the designated orphan use, irrespective of remaining patent life) for drugs that either treat conditions affecting fewer than 200,000 persons in the United States or that, in the FDA’s judgment, face market conditions making it unlikely that an innovator could recover its R&D costs. The Orphan Drug Act appears to have led to an increase in the number of new drugs for rare diseases. 49
Policies Affecting Generic Drugs. In addition to extending the period of market exclusivity on brand-name drugs, the Hatch-Waxman Act (enacted in 1984) also supports the development of generic drugs. It extends drug patents by up to five years but encourages competition from generic drugs once the patents on a pioneering drug have expired.
The legislation allows the FDA to approve most generic drugs without clinical trials. Instead, a manufacturer must show that its drug is pharmaceutically equivalent to the brand-name drug it copies, with the same active ingredients and no significant differences in the rate and extent of absorption at the site of drug action in the body.
The legislation also allows the FDA to extend by three years a brand-name drug’s market exclusivity for incremental changes, such as new indications, dosing regimens, or patient populations. (The FDA only grants that additional exclusivity when the manufacturer has conducted clinical trials that the agency judges were essential.) 50
Thus, the act strengthened incentives to develop new drugs by extending drug patent life, and it made it easier for lower-cost generic versions to be introduced when the drugs enter the public domain by allowing the FDA to approve most generics based on pharmaceutical equivalence rather than clinical trials.
Policies Affecting Biosimilar Drugs. Congress has sought to provide inducement to the development of biosimilar drugs—the analog, for biologic drugs, of the generic copies of small-molecule drugs. The Patient Protection and Affordable Care Act (P.L. 111-148) created an abbreviated pathway for FDA approval of biosimilar drugs. The manufacturer of a proposed biosimilar drug must demonstrate that the drug is “highly similar to and has no clinically meaningful differences from” the pioneering biologic drug. 51 In addition, biosimilar manufacturers do not need to conduct as many clinical trials as were conducted for the pioneering drug because they can cite the FDA’s safety and effectiveness determinations for the original biologic drug.
So far, that legislation has resulted in relatively few approved biosimilar drugs compared to the effect that the Hatch-Waxman Act had on the development of generic drugs. As of December 2020, the FDA had approved only 29 biosimilar drugs, and not all of them have been introduced. 52 Of the $125 billion in reported domestic retail spending on biologic drugs in 2017 (expressed here in 2019 dollars), $11 billion was spent on biologics for which biosimilar versions are available, and only $0.9 billion was spent on those biosimilars. 53
The relative lack of competition for pioneering biologic drugs might contribute to the shift in new-drug development toward biologic drugs instead of small-molecule drugs. In part, that shift might simply reflect advances in the underlying science. But biologic drugs are also attractive targets of research because they are harder to copy. The patent system does not require the original innovator to share the original cell line. Manufacturers seeking to make a biosimilar drug must develop their own living cell line to use as the basis for the new drug. By contrast, the primary challenge in making a generic copy of a small-molecule drug is to replicate the original drug’s active molecule, which is publicly disclosed in the patent. In addition, even under the abbreviated pathway specified by the FDA, biosimilar drugs must still be put through some clinical trials; unlike generic drugs, biosimilar drugs cannot avoid them altogether. 54
Biologic drugs may face less competition than small- molecule drugs. Independent of (but concurrent with) patent protection, the FDA grants pioneering biologic drugs 12 years of guaranteed exclusivity in contrast to 5 years of exclusivity for small-molecule drugs. 55 In addition, where biologic drugs are concerned, consumers may not as readily accept a biosimilar substitute as they do a generic drug, because a biosimilar is not identical to the drug it imitates. 56 Consumer acceptance may be increasing with greater availability and familiarity with biosimilars. However, certain federal payment policies and private contractual agreements may discourage the use of biosimilars. 57 With the possibility of facing less competition even beyond the period of market exclusivity, makers of biologic drugs would anticipate greater lifetime sales of those drugs as well.
Vaccine Policies . Several federal policies increase the demand for vaccines and therefore R&D spending to develop them. The federal Vaccines for Children program provides vaccines at no cost to children who might otherwise go unvaccinated because of their family’s inability to pay. Additionally, the Centers for Disease Control and Prevention publishes a schedule of recommended childhood and adult vaccinations, including specific recommendations for various groups, such as health care providers, travelers, expectant mothers, racial and ethnic populations, and people with certain underlying health conditions. Those recommendations induce individuals to have themselves and their children vaccinated, and federal subsidies lower the costs to consumers of those vaccinations. A study that analyzed the effects of such policies found that the recommendation in 1991 that infants be vaccinated against hepatitis B and the expansion of Medicare coverage to include the cost of influenza vaccination in 1993 were both associated with subsequent increases in the development of new vaccines. 58 Those findings suggest that manufacturers expected demand for vaccines to increase as a result of the new recommendations.
Federal policies also affect the supply of vaccines. The same study considered the federal Vaccine Injury Compensation Fund, which was established in 1986 to encourage manufacturers to develop and supply new vaccines by indemnifying the manufacturers against lawsuits arising from adverse reactions to childhood vaccines. The study found that the fund’s introduction was associated with increased development of new vaccines.
In 2020, the federal government invested directly in the development of vaccines by providing more than $19 billion in funding to support the private development of vaccines to prevent COVID-19 through its Biomedical Advanced Research and Development Authority (see Box 2 ).
Regulatory Policies . Federal regulatory policies that affect either drug supply or drug demand can influence drug companies’ returns on R&D spending, which would in turn affect the amount they were willing to spend on R&D. Proposed regulation of some drug prices would affect the sales volumes of existing drugs and, as a result, expected returns on R&D on future drugs; in turn, lower expected returns would result in fewer new drugs. Changes to regulation of clinical trials would also affect the supply of new drugs.
Drug Prices. U.S. markets are subject to less price regulation than are the markets in many other countries. Drug companies can mostly set their own prices, although some federal agencies purchase drugs at prices subject to a statutory cap, impose statutory limits on how quickly a manufacturer can raise its prices, or receive rebates from manufacturers that are specified in statute. 59
In 2019, the House of Representatives passed H.R. 3, which would have required the Secretary of Health and Human Services to negotiate with drug manufacturers over the domestic prices of certain high-priced, single-source drugs to ensure that they were no more than 20 percent higher than the average prices for those drugs in specific other countries. Under H.R. 3, drug manufacturers that did not agree to participate in negotiations or that failed to agree to a negotiated price would have been subject to an excise tax. The combination of income taxes and excise taxes on a drug’s sales might have caused the manufacturer to lose money if the drug were sold in the United States. Those taxes would have had the same effect as if the drug had not been approved for sale or as if there were a formulary—that is, a national list of drugs that insurers could cover—from which the drug was excluded. Therefore, the potential use of the excise tax would have served as a source of pressure on drug manufacturers in negotiations and would have lowered drug prices and federal spending, CBO estimated. 60 (For a discussion of the effects of lower prices on the introduction of new drugs, see Box 3 .)
More generally, state laws mandating or encouraging substitution of generic drugs for their brand-name equivalents help lower drug prices. 61 In addition, most Medicare Part D plans encourage the substitution of generic drugs for their brand-name equivalents. 62 And although the existence of generic drugs is enabled by the patent system’s disclosure requirement (compelling drugmakers to disclose the molecular structure of a drug’s active ingredient), several federal regulatory decisions hasten the introduction of those drugs. 63 Under the Hatch-Waxman Act, generic drugs shown to contain the same active ingredient as the pioneering drug do not need to be tested in clinical trials, as described above. The act also provides legal protections from claims of patent infringement to manufacturers who try to develop generic versions of a pioneering drug before its patents have expired and from liability for adverse events not listed on the label of the pioneering drug. 64
That competition from generic drugs—which can also reduce the demand for new drugs entering those markets—has tended to discourage investment in drug R&D. 65 Several studies have found that a real 10 percent decrease in the growth of drug prices would be associated with about a 6 percent decrease in pharmaceutical R&D spending as a share of net revenues. 66
Clinical Trials. A substantial R&D expense that can account for more than half of R&D spending (excluding capital costs), clinical trials are conducted in accordance with federal requirements. As a result, changes to federal policy regarding clinical trials can meaningfully affect private R&D spending. In particular, policymakers have made several changes to federal regulations governing clinical trials in an effort to reduce the time they take and therefore lower their cost.
For example, FDA’s guidance, described above, on how drug companies can establish bioequivalence between a biosimilar drug and the pioneering biologic drug is intended to minimize the expenses of clinical trials associated with developing biosimilar drugs. 67 The Prescription Drug User Fee Act, enacted in 1992, provided the FDA with additional resources to hasten the drug approval process, which reduced both the time to market and the capital costs of new-drug development.
More recently, federal policymakers have allowed the use of “surrogate endpoints” in drug trials for certain illnesses, including HIV infection and some cancers, to shorten some clinical trials. Surrogate endpoints include indirect, predictive indicators (such as blood pressure, cholesterol level, tumor size, T-cell counts, or other physical signs of disease), along with other test results and laboratory measures. 68 The FDA can approve certain kinds of drug for sale in the U.S. based on clinical-trials results that rely on such surrogate measures rather than on direct measures of a drug’s clinical benefit.
The use of surrogate endpoints has helped neutralize a tendency in privately funded research to emphasize treatments that can be commercialized more quickly, which can result in too little investment in clinically valuable treatments that would take longer to develop. 69 Speedier clinical trials can also benefit patients by hastening the introduction of life-extending therapies like the HIV antiretroviral treatments developed in the 1990s. 70 However, relying on surrogate endpoints means that consumers might spend money on some drugs that would turn out to have little clinically meaningful effect. 71
1 . See Pharmaceutical Research and Manufacturers of America, 2020 PhRMA Annual Membership Survey (PhRMA, 2020), https://tinyurl.com/ydh6p64t , and 2019 PhRMA Annual Membership Survey (PhRMA, 2019), https://tinyurl.com/ycvneve7 (PDF, 2.15 MB).
2 . The total includes only research funded by PhRMA member firms, including any contract research funded by those firms and performed on their behalf by universities or other contract-research laboratories. In particular, the PhRMA total does not include expenditures to acquire the R&D assets (such as drugs in development) of another firm.
3 . See National Science Foundation, “Business Enterprise Research and Development Survey” (accessed February 25, 2021), www.nsf.gov/statistics/srvyberd/ .
4 . See Pharmaceutical Research and Manufacturers of America, 2019 PhRMA Annual Membership Survey (PhRMA, 2019), Table 2, https://tinyurl.com/ycvneve7 (PDF, 2.15 MB) .
5 . That range applies to average R&D intensity for the approximately 4,000 firms in the Standard & Poor’s (S&P) Total Market Index, a combination of the S&P 500 Index and the S&P Completion Index (an index of the total U.S. stock market, excluding firms in the S&P 500). CBO chose the Total Market Index as a basis of comparison because of its breadth.
6 . See Congressional Budget Office, Prices for and Spending on Specialty Drugs in Medicare Part D and Medicaid (March 2019), www.cbo.gov/publication/54964 .
7 . Unobserved rebates are paid by manufacturers to insurers or buyers and are considered proprietary information.
8 . See Department of Veterans Affairs, “Hepatitis C Medications: An Overview for Patients” (accessed March 16, 2021), https://go.usa.gov/xs7qe .
9 . See IQVIA Institute for Human Data Science, Medicine Use and Spending in the U.S. (April 2018), p. 37, https://tinyurl.com/yd5cnvrl .
10 . See Qi Sun and Mindy Z. Xiaolan, “Financing Intangible Capital,” Journal of Financial Economics, vol. 133, no. 3 (September 2019), pp. 564-588, https://doi.org/10.1016/j.jfineco.2019.04.003 ; Bronwyn Hall and Josh Lerner, “ The Financing of R&D and Innovation ,” in Bronwyn H. Hall and Nathan Rosenberg, eds., Handbook of the Economics of Innovation , vol. 1 (North Holland, 2010), pp. 609–639; and Thomas W. Bates, Kathleen M. Kahle, and René M. Stulz, “Why Do U.S. Firms Hold So Much More Cash Than They Used To?” The Journal of Finance, vol. 64, no. 5 (October 2009), pp. 1985–2021, https://doi.org/10.1111/j.1540-6261.2009.01492.x .
11 . See R. Glenn Hubbard, “Capital-Market Imperfections and Investment,” Journal of Economic Literature, vol. 36, no. 1 (March 1998), pp. 193–225, www.jstor.org/stable/2564955 .
12 . See Government Accountability Office, Drug Industry: Profits, Research and Development Spending, and Merger and Acquisition Deals , GAO-18-40 (November 2017), p. 36, www.gao.gov/products/GAO-18-40 .
13 . Ibid., p. 37.
14 . A company can, within limits, influence its own success rate because that rate depends on the kinds of drugs the company chooses to pursue and to advance into clinical trials and on how the company manages its research process. For estimated success rates, see Chi Heem Wong, Kien Wei Siah, Andrew W Lo, “Estimation of clinical trial success rates and related parameters,” Biostatistics , vol. 20, no. 2 (April 2019), pp. 273–286, https://doi.org/ 10.1093/biostatistics/kxx069 ; David Thomas and others, Clinical Development Success Rates 2006–2015 (Biotechnology Innovation Organization, Amplion, and Biomedtracker, 2016), https://tinyurl.com/y2n8rnzb (PDF, 4.02 MB); and Michael Hay and others, “Clinical Development Success Rates for Investigational Drugs,” Nature Biotechnology , vol. 32, no. 1 (2014), pp. 40–51, https://doi.org/10.1038/nbt.2786 .
15 . See Joseph A. DiMasi, Henry G. Grabowski, and Ronald W. Hansen, “Innovation in the Pharmaceutical Industry: New Estimates of R&D Costs,” Journal of Health Economics, vol. 47 (May 2016), p. 25, https://doi.org/10.1016/j.jhealeco.2016.01.012 . The estimate reported in that study is $430 million in 2013 dollars.
16 . Ibid., p. 23.
17 . See Vinay Prasad and Sham Mailankody, “Research and Development Spending to Bring a Single Cancer Drug to Market and Revenues After Approval,” JAMA Internal Medicine, vol. 177, no. 11 (November 2017), pp. 1569–1575, https://doi.org/10.1001/jamainternmed.2017.3601 .
18 . See Barbara Bolten, “Fastest Drug Developers and Their Practices,” The CenterWatch Monthly, vol. 24, no. 8 (August 1, 2017), www.centerwatch.com/articles/13284%20 .
19 . See IQVIA Institute for Human Data Science, The Changing Landscape of Research and Development (April 2019), p. 7, https://tinyurl.com/y2kpxve8 .
20 . See Joseph A. DiMasi, Henry G. Grabowski, and Ronald W. Hansen, “Innovation in the Pharmaceutical Industry: New Estimates of R&D Costs,” Journal of Health Economics , vol. 47 (May 2016), pp. 23–24, https://doi.org/10.1016/j.jhealeco.2016.01.012 .
21 . Ibid., pp. 24–25. The corresponding values in the study, reported in millions of 2013 dollars, are $965, $25.3, $58.6, and $255.4, respectively.
22 . The values reported here all use a 7 percent cost of capital, as each study includes calculations that use that rate. (In its analysis of the budgetary effects of H.R. 3 for the 116 th Congress, CBO used an 8.1 percent cost of capital for drug R&D because that is CBO’s assessment of the cost; using a higher rate tends to slightly increase estimates of R&D costs.) See Congressional Budget Office, letter to the Honorable Frank Pallone Jr. regarding the budgetary effects of H.R. 3, the Elijah E. Cummings Lower Drug Costs Now Act (December 10, 2019), www.cbo.gov/publication/55936 . CBO has converted the values reported here to 2019 dollars.
23 . See Joseph A. DiMasi, Henry G. Grabowski, and Ronald W. Hansen, “Innovation in the Pharmaceutical Industry: New Estimates of R&D Costs,” Journal of Health Economics , vol. 47 (May 2016), p. 26–27, https://doi.org/10.1016/j.jhealeco.2016.01.012 . The values reported in the 2016 DiMasi study, in millions of 2013 dollars and using their central discount rate value of 10.5 percent, are $2,558, $1,098, and $1,460, respectively.
24 . Ibid., p. 20.
25 . For a critical review of the 2016 study by DiMasi and others, see Sammy Almashat, “Pharmaceutical Research Costs: The Myth of the $2.6 Billion Pill” (Public Citizen, September 2017), https://tinyurl.com/y4kb4xoq .
26 . See Christopher P. Adams and Van V. Brantner, “Estimating the Cost of New Drug Development: Is It Really $802 Million?” Health Affairs , vol. 25, no. 2 (March/April 2006), pp. 420–428, https://doi.org/10.1377/hlthaff.25.2.420 .
27 . See Olivier J. Wouters, Martin McKee, and Jeroen Luyten, “Estimated Research and Development Investment Needed to Bring a New Medicine to Market, 2009–2018,” Journal of the American Medical Association, vol. 323, no. 9 (2020), pp. 844–853, https://doi.org/10.1001/jama.2020.1166 . The study’s central published values differ from those reported above: they are expressed in 2018 dollars and use a 10.5 percent cost of capital. The authors also estimated R&D costs using a 7 percent discount rate.
28 . See Vinay Prasad and Sham Mailankody, “Research and Development Spending to Bring a Single Cancer Drug to Market and Revenues After Approval,” JAMA Internal Medicine, vol. 177, no. 11 (November 2017), pp. 1569–1575, https://doi.org/10.1001/jamainternmed.2017.3601 . The estimates reported in the study are in 2017 dollars.
29 . See Joseph A. DiMasi, Henry G. Grabowski, and Ronald W. Hansen, “Innovation in the Pharmaceutical Industry: New Estimates of R&D Costs,” Journal of Health Economics , vol. 47 (May 2016), p. 20, https://doi.org/10.1016/j.jhealeco.2016.01.012 . The estimate is based on the authors’ comparison of their 2016 findings with an estimate they published in 2007 ($1.2 billion, in 2005 dollars) using the same methods. See Joseph A. DiMasi and Henry G. Grabowski, “The Cost of Biopharmaceutical R&D: Is Biotech Different?” Managerial and Decision Economics , vol. 28, no. 4-5 (June–August 2007), pp. 469–479, https://doi.org/10.1002/mde.1360 .
30 . See Joseph A. DiMasi, Henry G. Grabowski, and Ronald W. Hansen, “Innovation in the Pharmaceutical Industry: New Estimates of R&D Costs,” Journal of Health Economics , vol. 47 (May 2016), Table 1, https://doi.org/10.1016/j.jhealeco.2016.01.012 .
31 . See Chi Heem Wong, Kien Wei Siah, and Andrew W. Lo, “Estimation of Clinical Trial Success Rates and Related Parameters,” Biostatistics , vol. 20, no. 2 (April 2019), pp. 273–286. https://doi.org/10.1093/biostatistics/kxx069 ; and Jorge Mestre-Ferrandiz, Jon Sussex, and Adrian Towse, The R&D Cost of a New Medicine (Office of Health Economics, United Kingdom, 2012).
32 . See Anup Malani and Tomas J. Philipson, Can Medical Progress Be Sustained? Implications of the Link Between Development and Output Markets , Working Paper 17011 (National Bureau of Economic Research, September 2012), www.nber.org/papers/w17011 .
33 . See Darius N. Lakdawalla, “Economics of the Pharmaceutical Industry,” Journal of Economic Literature, vol. 56, no. 2 (June 2018), p. 401, https://doi.org/10.1257/jel.20161327 .
34 . See Centers for Medicare & Medicaid Services, National Health Expenditures Data, “NHE Tables” (accessed December 16, 2020), Table 16, https://go.usa.gov/xASdV . In the table, the sum of expenditures by Medicare, Medicaid, and “Other Health Insurance Programs” (primarily the Veterans Health Administration, TRICARE, and the Children’s Health Insurance Program) accounts for 40 percent of total retail expenditures on prescription drugs in 2019.
35 . See Margaret E. Blume-Kohout and Neeraj Sood, “Market Size and Innovation: Effects of Medicare Part D on Pharmaceutical Research and Development,” Journal of Public Economics, vol. 97 (January 2013), pp. 327–336, https://doi.org/10.1016/j.jpubeco.2012.10.003 ; and David Dranove, Craig Garthwaite, and Manuel I. Harmosilla, Expected Profits and the Scientific Novelty of Innovation , Working Paper 27093 (National Bureau of Economic Research, May 2020), www.nber.org/papers/w27093 .
36 . For an analysis of likely effects of such a policy change on individuals’ decisions about health insurance and consumption of health-care services in general, see Congressional Budget Office, Options for Reducing the Deficit: 2019 to 2028 (December 2018), pp. 235–236, www.cbo.gov/publication/54667 .
37 . See Margaret E. Blume-Kohout, “Does Targeted, Disease-Specific Public Research Funding Influence Pharmaceutical Innovation?” Journal of Policy Analysis and Management , vol. 31, no. 3 (Summer 2012), pp. 641–660, https://doi.org/10.1002/pam.21640 ; and Michael R. Ward and David Dranove, “The Vertical Chain of Research and Development in the Pharmaceutical Industry,” Economic Inquiry, vol. 33, no. 1 (January 1995), pp. 70–87, https://tinyurl.com/z7huxuxv .
38 . See Kavya Sekar, National Institutes of Health (NIH) Funding, FY1995–FY2021, Report R43341, version 39 (Congressional Research Service, May 12, 2020), https://go.usa.gov/xshZu . Nominal funding levels have been adjusted for inflation by CBO using the gross domestic price index.
39 . Ekaterina Galkina Cleary and others, “Contribution of NIH Funding to New Drug Approvals 2010–2016,” Proceedings of the National Academy of Sciences , vol. 115, no. 10 (March 6, 2018), pp. 2329–2334. https://doi.org/10.1073/pnas.1715368115 .
40 . Department of Health and Human Services, Office of the Assistant Secretary for Planning and Evaluation, Report to Congress: Prescription Drug Pricing (May 20, 2020), https://go.usa.gov/xAVns (PDF, 2.04 MB).
41 . See Paul A. David, Bronwyn H. Hall, and Andrew A. Toole, “Is Public R&D a Complement or Substitute for Private R&D? A Review of the Econometric Evidence,” Research Policy , vol. 29, no. 4–5 (April 2000), pp. 497–529, https://doi.org/10.1016/S0048-7333(99)00087-6 ; and Bettina Becker, “Public R&D Policies and Private R&D Investment: A Survey of the Empirical Evidence,” Journal of Economic Surveys , vol. 29, no. 5 (December 2015), pp. 917–942, https:// doi.org/10.1111/joes.12074 .
42 . For additional information, see Wendy H. Schacht, Federal R&D, Drug Discovery, and Pricing: Insights From the NIH-University-Industry Relationship , Report RL32324 (Congressional Research Service, November 30, 2012).
43 . See Andrew A. Toole, “Does Public Scientific Research Complement Private Investment in R&D in the Pharma-ceutical Industry?” Journal of Law & Economics , vol. 50, no. 1 (February 2007), pp. 81–104, https://doi.org/10.1086/508314 .
44 . See Pierre Azoulay and others, “Public R&D Investments and Private-Sector Patenting: Evidence From NIH Funding Rules,” Review of Economic Studies , vol. 86, no. 1 (January 2019), pp. 117–15, https://doi.org/10.1093/restud/rdy034 .
45 . See Austan Goolsbee, “Does Government R&D Policy Mainly Benefit Scientists and Engineers?” American Economic Review , vol. 88, no. 2 (May 1998), pp. 298–302, www.jstor.org/stable/116937 .
46 . For example, only spending on research deemed to be “technological in nature” qualifies for the credit. See Congressional Budget Office, How Taxes Affect the Incentive to Invest in New Intangible Assets (November 2018), www.cbo.gov/publication/54648 .
47 . For a history and description of the credit, see Gary Guenther, Research Tax Credit: Current Law and Policy Issues for the 114th Congress, Report RL31181, version 70 (Congressional Research Service, June 18, 2016), https://go.usa.gov/xshBx .
48 . See Congressional Budget Office, How Taxes Affect the Incentive to Invest in New Intangible Assets (November 2018), www.cbo.gov/publication/54648.
49 . Wesley Yin, “Market Incentives and Pharmaceutical Innovation,” Journal of Health Economics, vol. 27, no. 4 (2008), pp. 1060–1077. https://doi.org/10.1016/j.jhealeco.2008.01.002 .
50 . See New Drug Product Exclusivity, 21 C.F.R. § 314.108 (2020).
51 . See Food and Drug Administration, “Biosimilar Development, Review, and Approval” (October 20, 2017), https://go.usa.gov/xASPs .
52 . See Food and Drug Administration, “Biosimilar Product Information” (December 17, 2020), https://go.usa.gov/xAVna .
53 . See IQVIA Institute for Human Data Science, Medicine Use and Spending in the U.S.: A Review of 2017 and Outlook to 2022 (April 2018), p. 11. https://tinyurl.com/y36l4bqt .
54 . See Food and Drug Administration, “Generic Drugs Undergo Rigorous FDA Scrutiny” (October 8, 2014), https://go.usa.gov/xAVRg , and “Biosimilar Development, Review, and Approval” (October 20, 2017), https://go.usa.gov/xAVR4 .
55 . For biologics, see 42 U.S.C. § 262(k)(7)(A) (2018); for orphan drugs, see 21 U.S.C. § 360cc (2018); for small-molecule drugs, see § 355(j)(5)(F)(ii) (2018). Companies can receive an additional six months of exclusivity (beyond its patent exclusivity) if a drug—in any of its formulations, dosages, or approved indications—is designed for pediatric patients. See Food and Drug Administration, “Qualifying for Pediatric Exclusivity Under Section 505A of the Federal Food, Drug, and Cosmetic Act: Frequently Asked Questions on Pediatric Exclusivity” (November 30, 2016), https://go.usa.gov/xAVRP .
56 . See Darius N. Lakdawalla, “Economics of the Pharmaceutical Industry,” Journal of Economic Literature, vol. 56, no. 2 (June 2018), pp. 403–404, https://doi.org/10.1257/jel.20161327 .
57 . See Revisions to Payment Policies under the Physician Fee Schedule and Other Revisions to Part B for CY 2018, 82 Fed. Reg. 52976, 53181 (November 15, 2017), www.govinfo.gov/app/details/FR-2017-11-15 ; and Tony Hagen, “Remove the Disincentives and Biosimilars Will Flourish,” The Center for Biosimilars (July 7, 2020), https://tinyurl.com/acq5f5t3 .
58 . See Amy Finkelstein, “Static and Dynamic Effects of Health Policy: Evidence From the Vaccine Industry,” Quarterly Journal of Economics, vol. 119, no. 2 (May 2004), pp. 527–564, https://doi.org/10.1162/0033553041382166 .
59 . See Congressional Budget Office, A Comparison of Brand-Name Drug Prices Among Selected Federal Programs (February 2021), www.cbo.gov/publication/56978 .
60 . See Congressional Budget Office, letter to the Honorable Frank Pallone Jr. regarding the budgetary effects of H.R. 3, the Elijah E. Cummings Lower Drug Costs Now Act (December 10, 2019), www.cbo.gov/publication/55936 ; Christopher Adams and Evan Herrnstadt, CBO’s Model of Drug Price Negotiations Under the Elijah E. Cummings Lower Drug Costs Now Act , Working Paper 2021-01 (Congressional Budget Office, February 2021), www.cbo.gov/publication/56905 .
61 . See Yan Song and Douglas Barthold, “The Effects of State-Level Pharmacist Regulations on Generic Substitution of Prescription Drugs,” Health Ecoomics, vol. 27, no. 11 (November 2018), pp. 1717-1737. https://doi.org/10.1002/hec.3796 .
62 . See Stacie B. Dusetzina and others, “Medicare Part D Plans Rarely Cover Brand-Name Drugs When Generics Are Available,” Health Affairs, vol. 39, no. 8 (August 2020), pp. 1326–1333, https://doi.org/10.1377/hlthaff.2019.01694 .
63 . The patent system enables imitation of innovation (such as generic copies of pioneering drugs) by requiring the innovator, in exchange for a patent on a pioneering drug, to disclose sufficient details about the invention to allow “a person having ordinary skill in the art” to replicate it when the patent expires. See 35 U.S.C. § 103 (2018).
64 . For legal protection against adverse-event liability, see Aaron S. Kesselheim, Jerry Avorn, and Jeremy A. Greene, “Risk, Responsibility, and Generic Drugs,” New England Journal of Medicine, vol. 367, no. 18 (November 1, 2012), pp. 1679–1681, https://doi.org/10.1056/NEJMp1208781 . In the Hatch-Waxman Act, those provisions are balanced by the provision of stronger patent protections to drug innovators, including extension of the statutory period of patent protection by a portion of the time the drug is under FDA review, and five years of ensured market exclusivity before the FDA may approve the first generic copy of a pioneering drug.
65 . See Joseph P. Cook, Graeme Hunter, and John A. Vernon, Generic Utilization Rates, Real Pharmaceutical Prices, and Research and Development Expenditures , Working Paper 15723 (National Bureau of Economic Research, February 2010), www.nber.org/papers/w15723 .
66 . See Carmelo Giaccotto, Rexford E. Santerre, and John A. Vernon, “Drug Prices and Research and Development Investment Behavior in the Pharmaceutical Industry,” Journal of Law and Economics , vol. 48, no. 1 (April 2005), pp. 194–214, https://doi.org/10.1086/426882 ; and F. M. Scherer, Industry Structure, Strategy, and Public Policy (Harper Collins, 1996).
67 . See Food and Drug Administration, “Bioavailability and Bioequivalence Studies Submitted in NDAs or INDs—General Considerations” (March 2014), https://go.usa.gov/xAV5f .
68 . For a comprehensive list of surrogate endpoints used, see Food and Drug Administration, “Table of Surrogate Endpoints That Were the Basis of Drug Approval or Licensure” (March 30, 2021), https://go.usa.gov/xASyF .
69 . See Eric Budish, Benjamin N. Roin, and Heidi Williams, “Do Firms Underinvest in Long-Term Research? Evidence from Cancer Clinical Trials,” American Economic Review, vol. 105, no. 7 (July 2015), pp. 2044–2085. https://doi.org/10.1257/aer.20131176 .
70 . See Mark G. Duggan and William N. Evans, “Estimating the Impact of Medical Innovation: A Case Study of HIV Antiretroviral Treatments,” Forum for Health Economics and Policy, vol. 11, no. 2 (January 2008), pp. 1–37, https://doi.org/10.2202/1558-9544.1102 .
71 . See Bishal Gyawali, Spencer Phillips Hey, and Aaron S. Kesselheim, “Assessment of the Clinical Benefit of Cancer Drugs Receiving Accelerated Approval,” JAMA Internal Medicine, vol. 179, no. 7 (May 28, 2019), pp. 906–913, https://doi.org/10.1001/jamainternmed.2019.0462 .
About This Document
This Congressional Budget Office report was prepared at the request of the Chairman of the Senate Committee on Finance. In accordance with CBO’s mandate to provide objective, impartial analysis, the report makes no recommendations.
David Austin and Tamara Hayford prepared the report with guidance from Joseph Kile, Lyle Nelson, and Julie Topoleski. Christopher Adams, Pranav Bhandarkar, and David Wylie (formerly of CBO) contributed to the analysis. Anna Anderson-Cook (formerly of CBO), Colin Baker, Paul Burnham, Julia Christensen, Michael Falkenheim, Sebastien Gay, Ryan Greenfield, Stuart Hammond, Evan Herrnstadt, Leo Lex, Paul Masi, John McClelland, Lara Robillard, Ellen Werble, Chapin White, and Katherine Young provided useful comments.
Pierre Azoulay of the Sloan School of Management at the Massachusetts Institute of Technology, Peter Bach of the Memorial Sloan Kettering Cancer Center, and Craig Garthwaite of the Kellogg School of Management at Northwestern University provided helpful comments on the draft. (The assistance of external reviewers implies no responsibility for the final product, which rests solely with CBO.)
Jeffrey Kling reviewed the report. The editor was Caitlin Verboon, and R. L. Rebach was the graphics editor and cover illustrator. The report is available on CBO’s website ( www.cbo.gov/publication/ 57025 ).
CBO continually seeks feedback to make its work as useful as possible. Please send any comments to [email protected] .

Phillip L. Swagel
- Open access
- Published: 08 May 2024
The digital transformation in pharmacy: embracing online platforms and the cosmeceutical paradigm shift
- Ahmad Almeman ORCID: orcid.org/0000-0002-6521-9463 1
Journal of Health, Population and Nutrition volume 43 , Article number: 60 ( 2024 ) Cite this article
437 Accesses
3 Altmetric
Metrics details
In the face of rapid technological advancement, the pharmacy sector is undergoing a significant digital transformation. This review explores the transformative impact of digitalization in the global pharmacy sector. We illustrated how advancements in technologies like artificial intelligence, blockchain, and online platforms are reshaping pharmacy services and education. The paper provides a comprehensive overview of the growth of online pharmacy platforms and the pivotal role of telepharmacy and telehealth during the COVID-19 pandemic. Additionally, it discusses the burgeoning cosmeceutical market within online pharmacies, the regulatory challenges faced globally, and the private sector’s influence on healthcare technology. Conclusively, the paper highlights future trends and technological innovations, underscoring the dynamic evolution of the pharmacy landscape in response to digital transformation.
Introduction
Digital technology is driving a massive shift in the worldwide pharmacy industry with the goal of improving productivity, efficiency, and flexibility in healthcare delivery. In the pharmacy industry, implementing digital technologies like automation, computerization, and robotics is essential to cutting expenses and enhancing service delivery [ 1 ]. With a predicted 14.42% annual growth rate, the digital pharmacy market is expanding significantly and is expected to reach a market volume of about $35.33 billion by 2026. This expansion reflects the pharmacy industry’s growing reliance on and promise for digital technologies [ 2 ].
Pharmacy services have always been focused on face-to-face communication and paper-based procedures. However, the drive for more effective, transparent, and patient-centered healthcare is clear evidence of the growing need for digital transformation. Breakthroughs like mobile communications, cloud computing, advanced analytics, and the Internet of Things (IoT) are reshaping the healthcare sector. These breakthroughs have the potential to greatly improve patient care and service delivery, as demonstrated in other industries including banking, retail, and media [ 3 ].
In the pharmacy industry, a number of significant factors are hastening this digital transition. Important concerns include the desire for cost-effectiveness, enhanced patient care, and more transparency and efficiency in medication development and manufacture. This change has been made even more rapid by the COVID-19 pandemic, which has highlighted the necessity for digital solutions to address the difficulties associated with providing healthcare in emergency situations [ 4 ].
In terms of specific technologies being adopted, artificial intelligence (AI) and machine learning are playing a pivotal role. The McKinsey Global Institute estimates that AI in the pharmaceutical industry could generate nearly $100 billion annually across the U.S. healthcare system. The use of AI and machine learning enhances decision-making, optimizes innovation, and improves the efficiency of research and clinical trials. This results in more effective patient care and a more streamlined drug development process [ 5 ].
The digital transformation in the pharmacy sector represents a pivotal shift in the delivery and experience of healthcare services. This evolution is more than a transient trend; it’s a fundamental alteration in the healthcare landscape [ 6 ]. The adoption of digital technologies is reshaping aspects of healthcare, including patient engagement and medication adherence, leading to enhanced healthcare outcomes. Research indicates that digital tools in pharmacy practices have resulted in more individualized and efficient patient care. Telehealth platforms, exemplified by companies like HealthTap, are being increasingly incorporated by pharmacies to augment patient care via technological solutions. The contribution of digital health technology to medication adherence is notable, employing a variety of tools such as SMS, mobile applications, and innovative devices like virtual pillboxes and intelligent pill bottles. These advancements are pivotal in addressing the critical issue of medication nonadherence in healthcare. Furthermore, digital health tools are empowering pharmacists with expanded clinical responsibilities, particularly in the management of chronic diseases like diabetes, where apps and smart devices provide essential features such as blood glucose tracking and medication reminders. This comprehensive integration of digital health into pharmacy practice signifies a transformative era in healthcare delivery and patient management [ 7 ].
Online platforms are being used increasingly by the pharmaceutical sector and educational institutions to improve efficiency, flexibility, and accessibility. The telepharmacy program at CVS Pharmacy is an example of how telepharmacy services, which provide remote counseling and prescription verification, bring pharmaceutical care to underprivileged communities [ 8 ]. Prescription accuracy and drug management are enhanced by e-prescribing software like Epic’s MyChart and digital health apps like Medisafe [ 9 ; 10 ]. Blockchain technology is also being investigated for transparent and safe supply chain management. Continuous learning and professional networking are made possible in education by Virtual Learning Environments (VLEs) like Moodle [ 11 ], simulation software like SimMan 3G Plus [ 12 ], Continuing Professional Development (CPD) platforms like the American Pharmacists Association [ 13 ], and online conference platforms, as shown in Fig. 1 . While these platforms offer significant benefits like enhanced access and cost-effectiveness, they also present challenges, including addressing the digital divide and ensuring the quality and credibility of online services to maintain professional standards and patient safety.
In this review, we summarized the digital transformation in the pharmacy sector, emphasizing the integration of online platforms and the emerging significance of cosmeceuticals. We discussed the global shift towards digital healthcare, including telehealth and online pharmacy services, and how these changes have been accelerated by the COVID-19 pandemic. The paper also examined the impact of digital technologies on pharmacy practice and education, with a focus on telepharmacy services, e-prescribing software, and digital health apps. Additionally, it addresses the challenges and opportunities presented by this transformation, including regulatory and safety concerns, and the need for continuous professional development in the digital era.
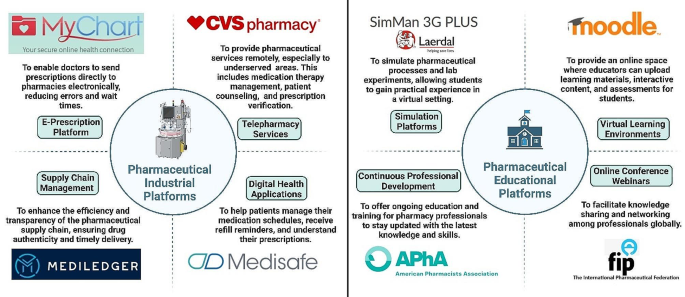
Comprehensive overview of different platforms in the pharmaceutical industry and education illustrating purposes and exemplary cases
The global impact of online pharmacy platforms
In recent years, the landscape of pharmacy practice and education has undergone a significant transformation, driven by technological advancements and catalyzed by the global COVID-19 pandemic. A study highlighting the increasing consumer trust in online medication purchases pre, during, and post-pandemic reveals a shift in consumer behavior towards online pharmacies [ 14 ]. This trend underscores a greater reliance on these platforms, where the perceived benefits significantly outweigh the perceived risks, indicating a positive reception and growing trust in digital healthcare solutions.
The adoption of telehealth, including telepharmacy, exemplifies this shift. In the United States, patient adoption of telehealth services surged from 11% in 2019 to 46%, with healthcare providers expanding their telehealth visits [ 15 ]. This shift is a reflection of how adaptable the healthcare sector is to digital platforms and how customer acceptance is increasing. The epidemic has also served as a catalyst, hastening the creation and uptake of online telepharmacy services throughout the world. The “new normal” has forced the addition of new platforms to support established sources of health information. The creation and evaluation of an online telepharmacy service in the Philippines during the pandemic serves as an example of this, demonstrating how quickly the global pharmacy industry adopted digital solutions. These services are essential for providing and elucidating pharmaceutical information within the context of primary healthcare delivery; they are not merely supplementary [ 16 ].
Simultaneously, pharmacist-led companies such as MedEssist and MedMehave, innovated digital platforms to facilitate services like flu shots or COVID-19 tests, reflecting a move towards customer-centric, digital-first services [ 17 ]. This transition enhances convenience and access to care but also introduces significant regulatory challenges. As the growth of online medicine sales disrupts traditional pharmacy markets, navigating these challenges becomes crucial for maintaining patient safety, quality standards, and fostering a trustworthy online healthcare environment [ 18 ].
Parallel to the practice changes, educational platforms for pharmacy have also evolved, especially under the impetus of the pandemic. These platforms have integrated a mix of traditional and student-centered teaching methodologies, including remote didactic lectures and on-site experiential training. The implementation of blended learning approaches, which combine remote lectures with on-site laboratory classes, reflects a broader educational trend towards hybrid models. This approach aims to leverage the advantages of both online and traditional methods, offering a more flexible and potentially more effective educational experience [ 19 ].
It takes more than just implementing new tools to integrate educational technology into pharmacy education, it also requires understanding how these technologies affect instruction and student learning. To effectively improve the educational experience, their utilization must have a purpose. There is an increasing amount of scholarly interest in this field, as evidenced by systematic reviews of the effects of new technologies on undergraduate pharmacy teaching and learning [ 20 ]. These digital platforms will probably become more significant in the future of pharmacy education, helping to mold the profession and guaranteeing that pharmacists are equipped to fulfill the ever-changing demands of the healthcare system. This development is indicative of a larger trend in the healthcare industry toward a more flexible, patient-focused, and technologically advanced environment [ 21 ].
Digital transformation in global healthcare
The recent advancements in digital transformation within global healthcare are significantly reshaping the landscape of healthcare and pharmacy services. These transformations are largely driven by the integration of digital technologies, which are redefining the tools and methods used in health, medicine, and biomedical science, ultimately aiming to create a healthier future for people worldwide [ 22 ]. In a 2018 report [ 23 ], Amazon’s potential entry into the $500 billion U.S. pharmacy market, the second-largest retail category, through mail-order and online pharmacies was highlighted as a significant industry disruptor. With licenses in at least 12 states in the US and a strategy focused on bypassing middlemen, Amazon’s historical success positions it to transform the pharmacy landscape, promising enhanced efficiency and cost savings for consumers.
One of the critical areas identified in recent research is the establishment of five priorities of e-health policy making: strategy, consensus-building, decision-making, implementation, and evaluation. These priorities emerged from stakeholders’ perceptions and are crucial for the effective integration and adoption of digital health technologies [ 24 ]. This holistic approach is increasingly relevant for scholars and practitioners, suggesting a focus on how multiple stakeholders implement digital technologies for management and business purposes in the healthcare sector [ 25 ]. The deployment of technological modalities, encompassed within five distinct clusters, can facilitate the development of a digital transformation model. This model ensures operational efficiency through several dimensions: enhanced operational efficacy by healthcare providers, the adoption of patient-centered methodologies, the integration of organizational factors and managerial implications, the refinement of workforce practices, and the consideration of socio-economic factors [ 25 ].
Studies focusing on value creation through digital means suggest healthcare as a consumer-centric realm ripe for center-edge transformations, characterized by self-service and feedback cycles. These transformations are vital in addressing inherent tensions between patients and physicians, steering the focus towards value co-creation and service-dominant logic [ 26 ]. Participatory design and decision-making approaches are emphasized for enhancing health information technology’s performance and institutional healthcare innovation. Such approaches are particularly crucial in developing national electronic medical record systems and improving chronic disease treatment through electronic health records. Additionally, telehealth research integrates patients’ perceptions, contributing to the understanding of technology, bureaucracy, and professionalism within healthcare [ 27 ].
The impact of health information technology (HIT) on operational efficiencies is profound. Empirical studies, such as those by Hong and Lee [ 28 ], Laurenza et al. [ 29 ], and Mazor et al. [ 30 ], demonstrate positive correlations between HIT and patient satisfaction, quality of care, and operational efficiency. However, challenges remain, as Rubbio et al. [ 31 ] highlight deficiencies in resilience-oriented practices for patient safety. Organizational and managerial factors in digital healthcare transformation also receive significant attention. Hikmet et al. [ 32 ] and Agarwal et al. [ 33 ] investigate the role of organizational variables and barriers in HIT adoption, whereas Cucciniello et al. [ 34 ] delve into the interdependence between implementing electronic medical records (EMR) systems and organizational conditions. Further, Eden et al. [ 35 ] and Huber and Gärtner [ 36 ] explore workforce adaptations and the implications of health information systems in hospitals that can increases transparency of work processes and accountability. Lastly, examining healthcare financialization and digital division provides an international perspective, contrasting the regulated environment in the EU with the US’s use of online medical crowdfunding as a potential solution to reduce bankruptcy [ 37 ; 38 ]. Collectively, these studies suggest a comprehensive model where stakeholders leverage digital transformation for management, enhancing operational efficiency in healthcare service providers.
Marques and Ferreira [ 39 ] performed a systematic literature review of digital transformation in healthcare, spanning the period from 1973 to 2018. Utilizing the SMARTER (Simple Multi-attribute Rating Technique Exploiting Ranks) method, 749 potential articles were analyzed, culminating in the prioritization and selection of 53 articles for detailed examination. The literature was organized into seven thematic areas: (1) Integrated management of IT in healthcare, (2) Medical images, (3) Electronic medical records, (4) IT and portable devices in healthcare, (5) Access to e-health, (6) Telemedicine, and (7) Privacy of medical data. It was observed that the predominant focus of research resides in the domains of integrated management, electronic medical records, and medical images. Concurrently, emerging trends were identified, notably the utilization of portable devices, the proliferation of virtual services, and the escalating concerns surrounding privacy. See Fig. 2 for visual representation of multifaceted digital transformation in healthcare.
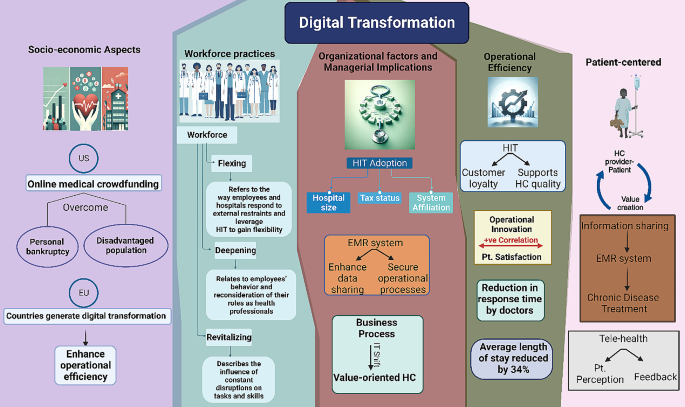
Visual representation of multifaceted digital transformation in healthcare: a synthesis of provider-patient dynamics, HIT impact, and strategic management. HIT; health information technology, HC; healthcare, EMR; electronic medical records. IT; information technology, Pt.; patient
Telehealth and online pharmacy advancements in pandemic management
In the realm of online pharmacies and telehealth, digital health technologies have been instrumental in managing the COVID-19 pandemic through surveillance, contact tracing, diagnosis, treatment, and prevention. These technologies ensure that healthcare, including pharmacy services, is delivered more effectively, addressing the challenges of accessibility and timely care. The role of telemedicine and e-pharmacies, in particular, has been emphasized in improving access to care worldwide. By enabling remote consultations and drug delivery, these platforms are making healthcare more accessible, especially in regions where traditional healthcare infrastructure is limited or overstretched [ 40 ].
The Canadian Virtual Care Policy Framework advocates for the swift adoption and integration of virtual care, propelled by the COVID-19 pandemic. It emphasizes enhancing access and quality, ensuring equity and privacy, and devising appropriate remuneration models, employing a collaborative, patient-centered approach while addressing digital disparities. During the COVID-19 pandemic, Canadian provinces and territories rapidly adopted virtual health care, leading to 60% of visits being virtual by April 2020, up from 10 to 20% in 2019. However, these implementations were often temporary and not fully integrated into healthcare systems. By August 2020, virtual visits decreased to 40%, with variations across regions, while provinces and territories used temporary billing codes for these services. The framework’s “Diagnostique” provides a thorough analysis of policy enablers and strategies for virtual care, underscoring the need for comprehensive policy and partnership engagement [ 41 ]. In the context of digital transformation in pharmacy, the Hospital News article outlines the application and infrastructure of telepharmacy services in Canada, highlighting the geographical challenges and the early adoption of telepharmacy in certain regions since 2003. It notes the use of various technologies like Medication Order Management, Videoconferencing, and Remote Camera Verification. Although lacking specific quantitative data, the article underscores the necessity for expanded telepharmacy services to ensure uniform care quality across diverse locations [ 42 ].
Similarly, Telehealth offers extensive resources for patients and providers in the United States, emphasizing programs like the Affordable Connectivity Program and Lifeline to facilitate access. The Health Resources and Services Administration enhances telehealth through support services, research, and technical assistance, reflecting a significant outreach impact [ 43 ]. The Office for the Advancement of Telehealth (OAT) under Health Resources and Services Administration (HRSA) works to improve access to quality health care through integrated telehealth services in the US. It supports direct services, research, and technical assistance, with over 6,000 telehealth technical assistance requests sent to Telehealth Resource Centers and approximately 22,000 patients served [ 44 ].
Internationally, In the UK, the National Health Service (NHS) spearheads digital health and care, providing significant innovation opportunities through vast data management. Support for digital health spans various stages, from discovery with organizations like Biotechnology and Biological Sciences Research Council (BBSRC) and Intelligent Data Analysis (IDA) research group, to development with networks such as Catapults and CPRD, and delivery with entities like the Academic Health Science Networks (AHSNs) and DigitalHealth.London. Regulatory bodies like the Medicines and Healthcare products Regulatory Agency (MHRA) and NICE ensure safety and efficacy. The collaborative ecosystem involves academic, healthcare, and industry stakeholders, aiming to enhance health and care services through technology and innovation [ 45 ].
In Australia, the government’s investment of over $4 billion into COVID-19 telehealth measures has facilitated universal access to quality healthcare. This initiative has provided over 85 million telehealth services to more than 16 million patients, with approximately 89,000 healthcare providers engaging in this service delivery. From 1 January 2022, telehealth services, initially introduced in response to COVID-19, will become an ongoing part of Medicare. This will allow eligible patients across Australia continued access to general practice (GP), nursing, midwifery, and allied health services via telehealth, deemed clinically appropriate by the health professional [ 46 ].
European nations such as the Netherlands, Austria, and Italy are at the forefront of implementing cross-organizational patient records, significantly enhancing telehealth communication and facilitating cross-border healthcare. The role of strong government support in advancing telehealth is pivotal. Ursula von der Leyen, the President of the European Commission, has been a prominent advocate for eHealth. She proposed the establishment of a European Health Data Space to streamline health data exchange across member states. France, a leader in telehealth legislation for nearly a decade, has pioneered a public funding scheme for tele-expertise at a national scale. Despite these advancements, challenges like legislative barriers and the lack of consistent political direction continue to impede progress in the telehealth domain [ 47 ].
The Asia-Pacific region anticipates a surge in telehealth adoption driven by digital demand and pandemic-induced behavioral changes, while South East Asia exhibits widespread telehealth growth across healthcare aspects [ 48 ]. The telehealth adoption across the Asia-Pacific region has shown remarkable growth between 2019 and 2021 and is projected to continue rising by 2024. China’s adoption nearly doubled to 47% and is expected to reach 76%. Indonesia’s usage more than doubled to 51%, with a forecast of 72%. Malaysia and the Philippines both anticipate reaching a 70% adoption rate, increasing from 30% to 29%, respectively. India’s adoption is projected to more than double to 68%, while Singapore, which had a significant increase from 5 to 45%, is expected to achieve a 60% adoption rate. This trend indicates a robust uptake of telehealth services in the region [ 48 ].
Global telemedicine and E-pharmacy policy dynamics
In the context of telemedicine and e-pharmacy regulations within South East Asia, a notable distinction emerges with Singapore, Malaysia, and Indonesia being the only countries to have formalized legal frameworks governing both telemedicine practices and the dissemination of electronic information. In these countries, tele-consultation is restricted to patients already under the care of healthcare practitioners or as part of ongoing treatment, specifically in Singapore and Malaysia. Additionally, for scenarios requiring more intensive medical intervention, such as new referrals, emergency cases, or invasive procedures, both Malaysia and Indonesia mandate physical presence and face-to-face consultations, emphasizing a cautious and regulated approach to remote healthcare. In Malaysia, the regulations further stipulate that online prescriptions, excluding narcotics and psychotropic substances, are permissible solely under the continuation of care model, reflecting a judicious use of digital prescription services [ 49 ].
In Central and Eastern Europe (CEE), telemedicine has experienced substantial growth, primarily catalyzed by the COVID-19 pandemic, which necessitated rapid advancements in technology and alterations in healthcare practices. The region’s robust digital infrastructure, coupled with the innovative drive of local companies and the challenges posed by an aging demographic, has significantly contributed to this expansion. According to the European Commission’s Market Study on Telemedicine, the global telemedicine market was projected to grow annually by 14% by 2021, a rate that was likely surpassed due to the pandemic’s impact. More specifically, the Europe Telehealth Market, valued at US $6,185.4 million in 2019, is anticipated to witness an annual growth rate of 18.9% from 2020 to 2030. This trend underscores the increasing reliance on and potential of telemedicine in addressing healthcare needs in the CEE region [ 50 ].
In the Middle East, telehealth and telepharmacy, have seen varied degrees of adoption and progress. Despite attempts to reform healthcare delivery in the region, the progress of telemedicine has been somewhat slow, with certain expectations yet to be fully realized. However, there has been notable development in the use and adoption of these technologies [ 51 ]. In a survey comparing the utilization of digital-health applications in Saudi Arabia and the United Arab Emirates (UAE), it was observed that a higher percentage of Saudi participants have utilized online pharmacy services (48%) compared to the UAE (36%). Conversely, awareness of teleconsultation services without prior use was higher in the UAE (43%) than in Saudi Arabia (35%). Retention data indicates that a significant proportion of users in both countries continue to engage with these services, with 80% of Saudi participants and 71% of UAE participants using teleconsultations at varying frequencies. Notably, a substantial majority of users in Saudi Arabia reported regular use of online pharmacies (90%), slightly higher than the UAE (78%), reflecting robust ongoing engagement with these digital health modalities. Notably, consumer adoption of telehealth products is primarily driven by time savings (48%) and convenience (47%), with 24-hour accessibility and efficacy both influencing 34% of users. Affordability and personal recommendations are also notable factors, while a wide range of options and quality are lesser but relevant considerations [ 52 ].
In response to the COVID-19 pandemic, a cross-sectional study was conducted among 391 licensed community pharmacists in the United Arab Emirates to assess the adoption and impact of telepharmacy services. The study revealed a predominant use of telepharmacy services, particularly via phone (95.6%) and messaging applications (80.0%). The findings highlighted that pharmacies with more pharmacists and those operating as part of a group or chain were more likely to implement a diverse range of telepharmacy services. The study identified significant barriers to telepharmacy adoption in individual pharmacies, including limited time, inadequate training, and financial constraints. There was a noticeable shift in service provision during the lockdown, with an increased reliance on telepharmacy, especially among pharmacies serving 50–100 patients per day. However, a reduction in services such as managing mild diseases and selling health products was observed during the lockdown period. The study concluded that telepharmacy played a pivotal role in supporting community pharmacies during the pandemic, with its expansion facilitated by the UAE’s advanced internet infrastructure, supportive health policies, and widespread digital connectivity [ 53 ]. Collectively, these insights reflect a global shift towards integrating and enhancing telehealth services as a response to emerging healthcare needs and technological advancements.
Unni et al. [ 54 ] provided an extensive review of telepharmacy initiatives adopted globally during the COVID-19 pandemic. Predominantly, virtual consultations were utilized to enable at-risk patients and others to remotely access pharmacists, thereby monitoring chronic illnesses, optimizing medication usage, and providing educational support [ 55 ]. Home delivery of medicines was widely implemented to decrease the necessity for in-person visits and mitigate exposure risks [ 56 ]. Additionally, patient education was prioritized to ensure effective management of health conditions from a distance [ 57 ]. Notably, a network of hospitals in China developed cloud-pharmacy care, allowing patients to consult pharmacists via text and the internet, while Spain utilized information and communication technologies for remote pharmaceutical care [ 58 ; 59 ]. Zero-contact pharmaceutical care, introduced in China, facilitated online medication consultations, eliminating direct contact [ 60 ]. The Kingdom of Saudi Arabia and other regions adapted new e-tools and teleprescriptions to enhance service accessibility [ 61 ]. The U.S. focused on credentialing pharmacists for telehealth to ensure competent service provision, and New Zealand implemented hotline numbers for phone consultations to further reduce physical visits [ 62 ; 63 ]. These initiatives reflect a significant shift towards innovative, technology-driven solutions in pharmaceutical care during a global health crisis. Refer to Fig. 3 for a graphical depiction of the worldwide distribution and applications of telepharmacy initiatives.
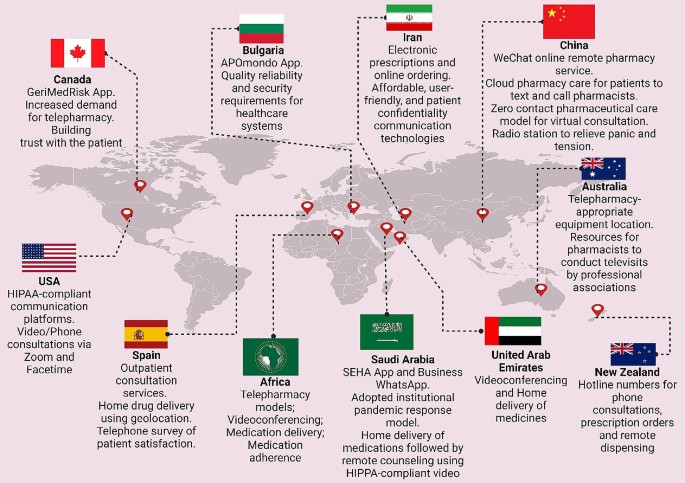
The global distribution of telepharmacy programs with an analysis of geographical distribution, technological applications, and associated benefits
Tracing the Private Sector’s Impact on Healthcare’s Technological Transformation
The role of the private sector in the fourth industrial revolution.
The World Economic Forum underscores the private sector’s leading role in digital inclusion and the acceleration of actions pertinent to the Fourth Industrial Revolution. This revolution affects economies, industries, and global issues profoundly, indicating the private sector’s critical role in driving technological advancements and digital platforms that deliver impactful healthcare solutions [ 64 ].
Mapping digital transformation in healthcare
A comprehensive analysis performed by Dal Mas et al. [ 65 ] meticulously maps the intricate terrain of digital transformation in healthcare, spotlighting the private sector’s instrumental role. Initially, the investigation encompassed an extensive array of diverse studies, leading to the identification of five main areas of digital technologies: smart health technologies, data-enabled and data collection technologies, Industry 4.0 tools and technologies, cognitive technologies, and drug & disease technologies. These domains frame the future research pathways, primarily steered by the private sector’s innovative drive. A significant proportion of the literature addresses healthcare broadly, suitable for both private and public sectors, yet a notable segment specifically focuses on the private sector’s endeavors, with a pronounced emphasis on the pharmaceutical domain [ 66 ; 67 ].
Public-private partnerships in healthcare delivery
The highlighted technologies, including digital platforms and telemedicine, exemplify the private sector’s trailblazing contributions to digital healthcare advancements. For instance, public-private partnerships (PPP) in India have emerged as a pivotal model for realizing universal healthcare (UHC), especially against the backdrop of acute healthcare shortages and urban-rural divides. Notably, mega PPP projects have successfully deployed technology-enabled remote healthcare (TeRHC), demonstrating its feasibility and impact in reaching isolated communities. These initiatives, overcoming various challenges, serve as a compelling example for global adoption, underscoring the transformative role of PPP in healthcare delivery [ 68 ].. Furthermore, a considerable majority of the literature in telemedicine underscores the necessity for profound research implications, yet a significant minority suggests policy implications [ 69 ; 70 ], reflecting a complex synergy between the private and public sectors in sculpting the digital healthcare framework [ 71 ]. This synthesis underscores the private sector’s critical influence in propelling the digital transformation in healthcare, charting a course that progressively fuses technological innovation with healthcare provision.
A study highlights Indonesia’s strategic initiatives to capitalize on telehealth business opportunities, driven by the Ministry of Research and Technology’s robust support for Technology-Based Start-up Company schemes [ 72 ]. With a demographic boon of 298 million from 2020 to 2024, escalating non-communicable diseases (71%), and a growing base of 222.4 million JKN participants, the stage is set for transformative growth. Despite a critical shortage of health workers (0.4 doctors per 1000 population), the enthusiasm for telemedicine is evident, with 71% satisfaction in hospital telemedicine and 32 million active telehealth users. The Ministry’s foresight in fostering technology start-ups, exemplified by the TEMENIN platform with its 11 health platforms, is steering Indonesia towards a future where high-quality healthcare is accessible and sustainable.
Lab@AOR: a model for PPPs in healthcare sector
The “Lab@AOR” initiative stands as a paradigmatic example of PPPs effectuating digital transformation within the healthcare sector. This strategic collaboration, between the University Hospital of Marche and Loccioni [ 73 ], a private entity, underscores the capacity of PPPs to navigate intricate challenges, stimulate international cooperation, and contribute to the development of sustainable, patient-centric healthcare solutions. Specifically, Lab@AOR was instituted to confront the nuanced challenges associated with the robotization of healthcare service delivery, highlighting the initiative’s role in fostering technological advancement through public and private sector synergy [ 74 ]. The project illustrates the evolution of Lab@AOR through three main phases: the pioneering stage, where groundwork for collaboration was laid; the nurturing stage, where collaborative exchanges were fostered; and the harvesting stage, wherein the potential of the PPP was fully unleashed. In the pioneering stage, Lab@AOR focused on a critical healthcare service component: the in-hospital preparation of medications for oncological patients. The University Hospital of Marche identified a need for innovation to improve service quality, efficiency, and safety, while Loccioni sought a real-life setting to test and refine its robotized system, APOTECAchemo [ 75 ]. This convergence of needs led to a symbiotic partnership aiming to enhance healthcare delivery through technological advancement.
During the nurturing stage, the partnership expanded the scope of APOTECAchemo to include non-oncological medications and developed additional tools like APOTECAps for manual preparation support. This phase was characterized by intensive collaboration, knowledge sharing, and continuous innovation, demonstrating the dynamic capability of the PPP to adapt and evolve in response to emerging healthcare challenges. The harvesting stage marked the international expansion of Lab@AOR, transforming it from a local initiative to an international community focused on leveraging digitalization and robotization to improve care quality and patient-centeredness. The PPP’s growth was catalyzed by its open perspective and inclusive approach, engaging entities from various cultural and institutional contexts, and fostering a network of 31 nodes across 19 countries and 3 continents.
Advancements in telehealth business models and frameworks
In their investigative study, Velayati et al. [ 76 ] delved into the articulation of emergent business models in telehealth and scrutinized the deployment of established frameworks across a variety of telehealth segments. The research spanned an extensive range of sectors, notably telemonitoring, telemedicine, mobile health, and telerehabilitation, alongside telehealth more broadly. The scope further extended to encompass niche areas such as assisted living technologies, sensor-based systems, and specific fields like mobile teledermoscopy, teleradiology, telecardiology, and teletreatment, presenting a thorough analysis of the telehealth landscape. Within the telemedicine and telehealth services sector, Barker et al. [ 77 ] introduced the Arizona Telemedicine Program (ATP) Model, a quintet-layer approach aimed at efficiently distributing telemedicine services throughout Arizona. Complementing this, Lee and Chang [ 78 ] proposed a four-component model specifically tailored for mobile health (mHealth) services pertaining to chronic kidney disease, focusing on offering a cost-effective platform for disease support and management. In the realm of telemonitoring, Dijkstra et al. [ 79 ] utilized the Freeband Business Blueprint Method (FBBM), which includes service, technological, organizational, and financial domains, to facilitate multiple telemonitoring services. Furthermore, the systemic and economic differences were explored in care coordination through Business to customer (B2C) and business (B2B) models for telemonitoring patients with chronic diseases, with the B2C model’s economic advantages were highlighted [ 80 ].
General telemedicine frameworks also received attention. Lin et al. [ 81 ] constructed a six-component framework analyzing major telemedicine projects in Taiwan, while Peters et al. [ 82 ] developed the CompBizMod Framework in Germany, encompassing value proposition, co-creation, communication and transfer, and value capture, designed to evaluate and enhance competitive advantages in telemedicine. In the specialized field of telecardiology, a comprehensive nine-component sustainable business model was crafted to facilitate mutual benefits for service providers and patients. This model emphasizes the importance of a holistic approach in ensuring the longevity and effectiveness of healthcare delivery within this domain [ 83 ]. Meanwhile, Mun et al. [ 84 ] presented a suite of five teleradiology business models aimed at providing effective, high-quality, and cost-efficient diagnoses.
The teletreatment sector saw innovative models from Kijl et al. [ 85 ], who designed a model for treating patients with chronic pain, focusing on the interrelation of components in the value network and the role of information technology. Complementarily, Fusco and Turchetti [ 86 ] introduced four models for telerehabilitation post-total knee replacement, emphasizing partnerships between care units and equipment suppliers to reduce costs and waiting lists. The mHealth and assisted living technology sector witnessed the introduction of a wearable biofeedback system model by Hidefjäll and Titkova [ 87 ], which employed Alexander Osterwalder’s Business Model Canvas and focused on a comprehensive commercialization process. Additionally, Oderanti and Li [ 88 ] presented a seven-component sustainable business model for assisted living technologies, aimed at encouraging older individuals to invest in eHealth services while reducing the pressure on health systems. These diverse clusters and models reflect the multifaceted nature of telehealth, each tailoring its approach to meet the unique demands of its domain. They collectively aim to optimize service delivery, stakeholder involvement, cost efficiency, and patient care quality, marking significant strides in the ongoing evolution of digital healthcare.
Challenges and biases in healthcare technology
One key aspect is the emergence of novel medical technologies and their potential biases. These biases are often a result of insufficient consideration of patient diversity in the development and testing phases. For example, disparities in the performance of medical devices like pulse oximeters among different racial groups have been observed, potentially due to a lack of diverse representation in clinical trials. This indicates a tendency for the development of healthcare technologies that may not adequately serve all patient populations [ 89 ]. A study on the profitability and risk-return comparison across health care industries highlights the use of return on equity (ROE) as a measure of profitability from a shareholder’s perspective. This measure combines profit margin, asset utilization, and financial leverage. The study analyzed financial data of publicly traded healthcare companies, providing insights into the financial dynamics of the healthcare sector. It revealed that while companies like Pfizer Inc. and UnitedHealth Group reported similar profitability, they had substantial differences in profit margin and asset utilization, indicating diverse financial strategies within the healthcare sector. This study underscores the complexity of financial performance in healthcare, where profitability measures need to be balanced with risk assessment and the broader impact on healthcare provision [ 90 ].
Additionally, an article discusses the benefits, pitfalls, and potential biases in healthcare AI. It emphasizes that as the healthcare industry adopts AI, machine learning, and other modeling techniques, it is seeing benefits for both patient outcomes and cost reduction. However, the industry must be mindful of managing the risks, including biases that may arise during the implementation of AI. Lessons from other industries can provide a framework for acknowledging and managing data, machine, and human biases in AI. This perspective is crucial in understanding how the integration of advanced technologies in healthcare can be influenced by the drive for profitability and efficiency, possibly at the expense of equitable and patient-centered care [ 91 ; 92 ].
Cosmeceuticals in the online pharmacy market
Cosmeceuticals, a term derived from the combination of cosmetics and pharmaceuticals, refer to a category of products that are formulated to provide both aesthetic improvements and therapeutic benefits. These products, typically applied topically, are designed to enhance the health and beauty of the skin, going beyond the mere cosmetic appearance. The exploration of cosmeceuticals in the online pharmacy market reveals a multifaceted and rapidly expanding industry. Bridging the gap between cosmetics and pharmaceuticals, they form a significant portion of the skincare industry. Cosmeceuticals are formulated from various ingredients, with their main categories being constantly discussed and analyzed in the scientific community [ 93 ]. They have taken a considerable share of the personal care industry globally, constituting a significant part of dermatologists’ prescriptions worldwide [ 94 ]. This surge is further fueled by increasing consumer demand for effective and safe products, including anti-aging skincare cosmeceuticals, a need which has been intensified by concerns over pollution, climate change, and the COVID-19 pandemic [ 95 ].
The global cosmeceuticals market is experiencing robust growth. Valued at USD 56.78 billion in 2022, it’s projected to expand to USD 95.75 billion by 2030, with a compound annual growth rate (CAGR) of 7.45%. This growth trajectory is propelled by the innovative integration of bioactive ingredients known for their medical benefits [ 96 ]. Another report confirms this upward trend, indicating the market was worth $45.56 billion in 2021 and is on a path of significant growth to USD 114 billion by 2030. The global disease burden is significantly impacted by various skin diseases, with dermatitis, psoriasis, and acne vulgaris among the most prevalent, contributing 0.38%, 0.19%, and 0.29% respectively. The pervasive nature of these conditions drives a substantial demand for effective treatments, propelling the integration of cosmeceuticals into the online pharmacy market. This integration not only offers convenient access to a range of therapeutic skincare products but also caters to the rising consumer inclination towards self-care and preventive healthcare. As a result, the online availability of cosmeceuticals is not just addressing the immediate needs of individuals suffering from skin conditions but is also reshaping the landscape of personal healthcare by making specialized treatments more accessible and customizable [ 97 ]. See Fig. 4 .
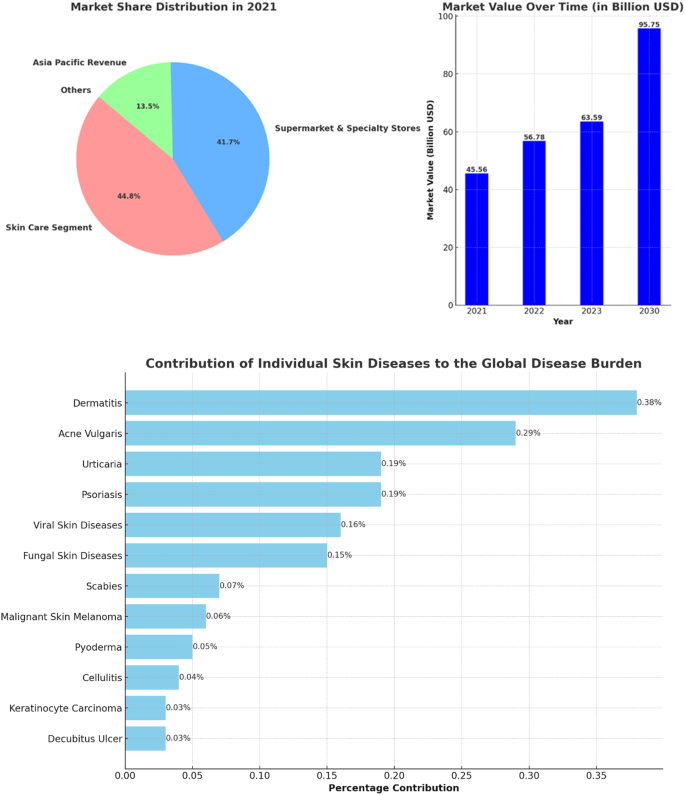
The left panel presents the market share distribution for key segments in the cosmeceuticals industry in 2021, including Skin Care Segment, and Supermarket & Specialty Stores, for Asia Pacific Revenue, with percentages for each category. The right panel displays the market value progression over time from 2021 to the projected value in 2030, with bold numbers indicating the value in billion USD for each year. The lower horizontal bar chart depicts the percentage contribution of various skin diseases to the global disease burden
Several factors are contributing to this expansion of the cosmeceuticals market. The market is driven by innovation in natural ingredients and a significant penetration of internet, smartphone, and social media applications, which attract potential consumer populations and reflect constantly changing consumer behavior [ 98 ]. The cosmeceuticals market’s robust CAGR and revenue share, especially in regions like Asia Pacific, further signify its burgeoning presence and potential within the global market [ 99 ]. Integration into online pharmacies is a key aspect of this market’s evolution, offering easier access to these products for a wider customer base. As the market continues to grow, it’s anticipated that the blend of cosmeceuticals with online pharmaceutical platforms will become increasingly seamless, offering consumers a diverse range of accessible, effective, and beneficial skincare and health products. This integration is likely to be driven by the growing trend of e-commerce and digitalization in healthcare and personal care sectors.
The landscape of online pharmacies, particularly concerning cosmeceuticals, is evolving. While the overall penetration for non-specialty drugs in mail-order and online pharmacies is low, they represent a significant portion of specialty prescription revenues at 37%. Despite this, only 13% of consumers consider these as their primary pharmacy choice, indicating a growing but still emerging market. Strategies are in place to enhance the market appeal of these pharmacies, focusing on speed, convenience, and personalized experiences, such as video telehealth visits, to attract a broader consumer base [ 100 ].
The dissertation “L’Oréal Portugal: A Digital Challenge for the Active Cosmetics Division” authored by Ascenso [ 101 ] provides an in-depth examination of the impact of digital evolution on the Portuguese cosmeceutical sector and its implications for L’Oréal, a significant cosmetics company. It posits that while L’Oréal has foundational digital competencies, the rapidly evolving digital landscape presents a broad spectrum of potential risks and opportunities. The study details the operations of L’Oréal’s Active Cosmetics Division, which manages brands predominantly sold in pharmacies and parapharmacies, and explores the potential repercussions of digitalization on L’Oréal Portugal’s strategic and operational frameworks. Furthermore, the thesis highlights the expanding role of e-pharmacies and the need for legal reforms to facilitate their operation. It discusses the prevalent trends in the cosmetic industry, such as the increasing demand for natural, male-focused, and environmentally friendly products. The dissertation scrutinizes L’Oréal’s strategic pillars, including innovation, acquisition, and regional growth, emphasizing the need for the company to integrate advanced technologies and recalibrate its business methodologies in light of digital progression [ 101 ]. Although L’Oréal has initiated some digital strategies targeting consumers and pharmacies, there’s a recognized need for an intensified focus on digital marketing aimed at clients. An exploratory attempt by L’Oréal to implement an online ordering platform for pharmacies did not meet success, indicating possible industry unreadiness for such advancements. This case study serves as a critical examination of how traditional companies in the pharmaceutical and cosmetics sectors must adapt to the digital age’s challenges and opportunities [ 101 ].
In a collaborative endeavor with L’Oréal, an associated digital agency provided a comprehensive suite of services that encompasses the full management of social media pages, the development of e-commerce websites, the establishment of Customer Relationship Management (CRM) platforms tailored for pharmacies, and the execution of digital campaigns leveraging QR codes, SMS marketing, and newsletters. These digital tools confer a competitive edge, facilitating a deeper comprehension of consumer behavior and the potential to augment value extraction from customer interactions. For the laboratories, particularly those associated with cosmetics, the advantages are twofold: an increase in sell-out figures, thereby enhancing direct sales to end consumers, and a boost in sell-in metrics, reflecting a rise in transactions to pharmacies or wholesalers. The online ordering feature, as noted by João Roma, a manager at La Roche-Posay, could result in a cacophony of processes if laboratories were to individually develop distinct methods. He advocates for the utilization of pre-existing platforms, such as the established e-learning infrastructure, to spearhead ventures into the online marketplace [ 101 ].
A survey conducted specifically for L’Oréal’s e-learning platform, cosmeticaactiva.pt [ 102 ], across the Portuguese landscape garnered responses from 324 participants, comprising 71% general pharmacists, 13% technical assistants, 8% directors, 7% individuals responsible for procurement from laboratories, and 2% beauty/cosmetic advisors. The findings from this survey underscore the pervasive adoption of digital tools within the pharmacy sector: 82% of respondents affirmed the presence of their pharmacies on social media platforms, 80% reported the use of basic management software, 64% indicated the deployment of advanced management systems, 61% were conversant with online ordering systems directed at laboratories, 38% utilized a store locator, 28% had an established website presence, and a smaller segment of 12% offered online shopping facilities.
Another survey conducted within this study to evaluate the significance of dermocosmetic products in pharmacies yielded a mean importance rating of 4.38 out of 5, indicating that a majority of pharmacists consider these products to be highly important to their business operations. Factors critical to the differentiation of a proficient laboratory/supplier were innovation and cost-effectiveness, with mean scores of 1.9 and 2.7 respectively, on a scale from 1 (most important) to 5 (least important). A substantial majority of pharmacists, amounting to 81.8%, perceive their pharmacies as beacons of innovation and modernity. Detailed interviews elucidated that digital tools are indispensable in augmenting sales for cosmeceutical products by catalyzing demand—a dynamic not feasible with medicinal products. These tools are paramount in managing customer loyalty, facilitating enhanced communication with existing clients via online and mobile channels. Despite the challenges posed by digitalization, particularly in the realms of logistics and human resources, the management at L’Oréal is well-equipped to swiftly adapt to the evolving business landscape, as evidenced by the proactive adoption and integration of these digital strategies [ 101 ] as illustrated in Fig. 5 .
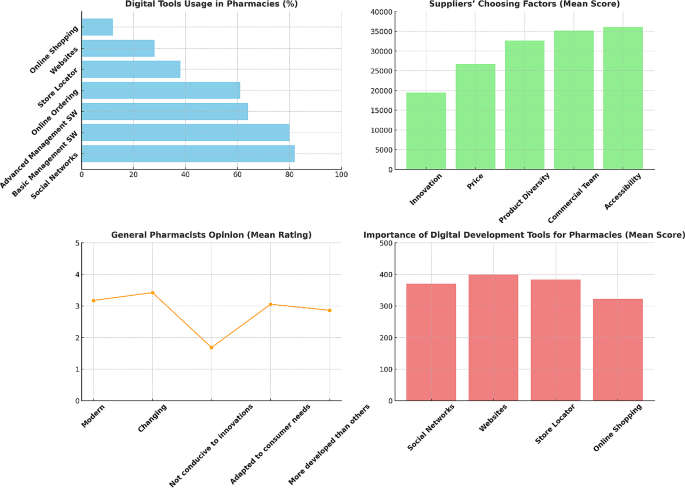
Results from Ascenso [ 101 ] survey assessing digital challenges for L’Oréal in the Portuguese cosmeceutical sector. Digital Tools Usage in Pharmacies (upper left) : the bar chart showing the percentage of respondents using various digital tools in pharmacies. Suppliers’ Choosing Factors (upper right) : the bar chart displaying the mean scores of factors that distinguish a good laboratory/supplier. General Pharmacists Opinion (lower left) : A line chart illustrating the mean ratings of pharmacists’ opinions on whether the pharmaceutical sector is modern, changing, conducive to innovations, adapted to consumer needs, and more developed than other sectors. Importance of Digital Development Tools for Pharmacies (lower right) : A vertical bar chart demonstrating the mean scores for the importance of different digital development tools for pharmacies
The digital transformation strategies, exemplified by companies like L’Oréal, extend beyond the mere targeting of end consumers, encompassing the perspectives of various stakeholders, including retailers. This broadened focus reflects a holistic and integrated approach to digital marketing and customer engagement, indicative of a larger trend within the market. The significance of digital channels in facilitating comprehensive customer interaction and brand development is increasingly recognized. The distinction of organizations such as L’Oréal in their digital initiatives highlights the competitive advantage that can be garnered through innovative digital strategies.
The receptiveness of industry professionals, such as pharmacists, to emerging digital trends, along with the readiness of companies to engage in non-face-to-face sales models, marks a paradigm shift in traditional sales and distribution methods. This shift is reflective of a broader market trend where digital platforms are becoming integral to the customer journey. Furthermore, the potential for online sales in specialized sectors, such as dermocosmetics, and the benefits that organizations derive from the technological advancement of their client base, underscore an escalating acknowledgment of e-commerce and digital tools as crucial elements of a business strategy. This trend, with L’Oréal as a prime example, emphasizes the broader market movement towards digital transformation, not merely as an option but as a necessity for maintaining relevance and competitiveness in an ever-evolving market landscape.
The global regulatory landscape for cosmeceuticals
Sophisticated regulatory legislation and enforcement mechanisms characterize many developed countries such as the USA, EU Member States, Canada, and Japan. These nations, along with influential organizations like the World Health Organization (WHO), significantly shape international market rules and regulations due to their market size and regulatory capacity [ 103 ]. The WHO is particularly noted for its crucial role in setting global standards, with a focus on developing and promoting international standards related to food, biological, pharmaceutical, and similar products [ 104 ]. In contrast to pharmaceuticals, the cosmetic industry necessitates a more advanced international regulatory framework due to consumers’ extensive exposure to these products. The distinction between cosmetics and pharmaceuticals varies significantly across different countries, with the USA employing a voluntary registration system for cosmetics and the EU and Japan requiring mandatory product filings prior to marketing [ 105 ]. Concerns over the safety of pharmaceutical and cosmetic products are highlighted, with an increasing consumer focus on “natural, ecological, and clean” products [ 106 ]. However, the lack of a regulatory framework for these categories underscores the need for more advanced regulations to mitigate health risks.
Intergovernmental cooperation is emphasized, with the US and EU portrayed as dominant players in the pharmaceutical and cosmetic industries, respectively. Regulatory capacity, which is essential for defining, implementing, and monitoring market rules, varies among countries and markets. This capacity depends on several factors, including staff expertise, statutory sanctioning authority, and the degree of centralization of regulatory authority [ 103 ]. The regulatory systems of the EU and US are explored, focusing on their unique approaches to medicine authorization and regulation. The European Medicines Agency (EMA) in the EU and the Food and Drug Administration (FDA) in the US serve as pivotal regulatory bodies [ 107 ; 108 ]. The EMA’s centralized procedure and the FDA’s premarket approval process are detailed, along with subsequent postmarket regulatory procedures. For instance, EU and US cosmetic regulations are compared, revealing differences in their approaches and the evolution of the EU’s regulatory landscape through various amendments and directives. In particular, directive 76/768/EC has been superseded by Regulation (EC) N° 1223/2009, serving as the principal regulatory framework for finished cosmetic products in the EU market. This regulation enhances product safety, optimizes the sector’s framework, and eases procedures to promote the internal cosmetic market. Incorporating recent technological advancements, including nanomaterials, it maintains an internationally acknowledged regime focused on product safety without altering existing animal testing prohibitions [ 109 ].
The Eurasian Economic Union’s (EAEU) regulatory framework for medicines and medical devices is detailed, including the legal framework established for regulating the circulation of these products. The conformity assessment methods, such as the EAC Declaration and the State Registration process, are required for manufacturers to demonstrate their products’ compliance with the standards [ 110 ]. Armenia is also part of the EAEU’s legal framework, which aims to unify regulations for the production and registration of pharmaceuticals and medical products by 2025. This unification is expected to reduce administrative costs for manufacturers and improve medicinal products for patients. Despite significant developments in the cosmetics industry, Armenia does not have an extensive regulatory framework for it. Prior to joining the EAEU, the only regulation concerning cosmetic products was the Order of the Minister of Health of the Republic of Armenia on “Hygiene Requirements of the Production and Safety of Perfume-Cosmetic Products.” Since joining the EAEU, Armenia has unified its national legislation with EAEU regulations, but there are challenges and gaps in the direct applicability of the EAEU’s technical regulations in the country [ 111 ].
In the context of the necessity for clear regulatory framework stems from two reasons. Firstly, cosmeceuticals - products straddling cosmetics and drugs - demand intensified regulatory attention. Examples include the 2007 FDA seizure of Jan Marini’s Age Intervention Eyelash, which contained the drug ingredient bimatoprost, and products boasting human stem cell cultured media, which claim rejuvenating effects but may pose safety risks due to minimal oversight [ 112 ]. A noted 1450% increase in FDA warnings (from 4 to 62 letters) between 2007 and 2011 and 2012–2017, with 8 targeting stem cell ingredient promotions, underscores the growing concern [ 113 ]. The FDA’s limited capacity to identify and assess potential drug-adulterated cosmetics raises concerns.
The second aspect focuses on the necessity for a more comprehensive and unbiased scientific and medical perspective in the FDA’s ingredient review process. The Personal Care Products Safety Act proposes a balanced committee formation including industry, consumer, and medical representatives, yet advocates for the inclusion of specialized professionals like chemists, dermatologists, toxicologists, and endocrinologists. Specific ingredients like diazolidinyl urea and quarternium-15, although effective antimicrobials, are flagged for potential skin allergy risks and formaldehyde release. The preservative 4-methylisothiazolinone, banned in Europe for rinse-off products, is noted for increasing allergic contact dermatitis cases in the US [ 114 ]. The lag in US cosmetic regulation compared to the EU is acknowledged, with the Personal Care Products Safety Act considered a significant advancement, albeit in need of further refinement [ 115 ].
The importance of consumer safety in the global regulatory landscape for cosmeceuticals, particularly for products that blur the line between cosmetics and pharmaceuticals, is a critical issue due to several key factors. Firstly, the cosmeceutical market is expanding rapidly, driven by new ingredients promising various skincare benefits like anti-aging and photoprotection. This growth necessitates clear regulatory guidelines to ensure that these products are safe and their claims are clinically proven. The FDA, for instance, differentiates between cosmetics and cosmeceuticals based on their intended use, particularly if a product is marketed as a cosmetic but functions in a way that affects the structure of the human body, classifying it as a cosmeceutical [ 116 ].
Secondly, the legal and regulatory distinctions between drugs and cosmetics are significant. Drugs are subject to FDA approval based on their intended use in treating diseases or affecting the body’s structure or function, whereas cosmetics are not. This difference becomes crucial when products are marketed with drug-like claims but are not regulated as drugs, potentially leading to consumer safety issues. For example, botanical cosmeceuticals, which contain natural ingredients like herbal extracts, need thorough evaluation to ensure consistency in therapeutic effects [ 117 ]. Additionally, cosmeceutical manufacturers must be careful with marketing and advertising claims to avoid legal implications. Misleading claims can lead to lawsuits and regulatory actions, as seen in past cases where companies faced consequences for unfounded product claims. Moreover, the FDA advises cosmeceutical manufacturers to follow Good Manufacturing Practices (GMP) to reduce the risk of misbranding or mislabeling. These guidelines include production practices and specific warning statement guidelines, emphasizing the importance of substantiating the safety of these products [ 118 ].
The global regulatory landscape for online pharmacy
Online pharmacies pose various risks to consumers, including the potential health hazards from counterfeit or substandard medications and the inappropriate use of prescription drugs. The regulatory landscape for these pharmacies varies significantly across nations, with some countries like the United States implementing specific laws, while others, such as France, have instituted outright bans [ 119 ]. The European Union, for instance, has implemented a mandate effective from 1 July 2015, which requires member states to adhere to legal provisions for a common logo specific to online pharmacies. This is coupled with an obligation for national regulatory authorities to maintain a registry of all registered online medicine retailers, as detailed by the European Medicines Agency [ 120 ]. Furthermore, the sale of certain medications online within the EU is permissible, contingent upon the registration of the pharmacy or retailer with respective national authorities [ 121 ]. Additionally, the Council of Europe’s MEDICRIME Convention introduces an international treaty that criminalizes the online sale of counterfeit medicinal products, enforcing prosecution irrespective of the country in which the crime is perpetrated [ 122 ].
Switzerland presents a unique stance, where Swissmedic strongly advises against the online purchase of medicines due to the high risk of illegal sourcing and poor quality. However, Swiss mail-order pharmacies with a valid cantonal license to operate a mail-order business are exempted from this advisory [ 123 ]. The Swiss Mail-Order Pharmacists Association and its affiliates, such as Zur Rose AG and MediService AG, actively advocate for a modern and equitable regulation of mail-order medicine sales [ 124 ]. The legislative framework is further bolstered by the Federal Act on Medicinal Products and Medical Devices, which regulates therapeutic products to guarantee their quality, safety, and efficacy [ 125 ]. In the Middle East, community pharmacy practice is predominantly governed by national Ministries of Public Health or equivalent governmental entities, with most community pharmacies being privately owned [ 126 ]. The region’s involvement in the Global Cooperation Group, which encompasses various international regulatory bodies like the EMA and USFDA, signifies a collaborative approach towards drug regulatory affairs in the MENA region [ 127 ]. Despite these advances in regulatory collaboration, it is notable that currently no specific regulations have been detected for online purchases from online pharmacies in the Middle East, highlighting a significant area for potential regulatory development. Furthermore, a notable transition is observed in pharmacy education across several Middle Eastern nations, with an inclination towards introducing Pharm.D degrees to replace traditional pharmacy degrees, reflective of evolving educational standards in the pharmaceutical field [ 128 ]. This shift in education parallels the need for updated regulatory frameworks, especially in the context of the burgeoning online pharmacy sector.
Furthermore, Australia permits the sale of both Prescription-Only Medicines (POMs) and Over-the-Counter (OTC) medications online, provided that brick-and-mortar pharmacies comply with all relevant laws and practice standards [ 129 ]. In contrast, South Korea maintains a stringent stance, prohibiting the online sale of both POMs and OTC medicines, with sales confined exclusively to physical stores registered with the Regulatory Authority (RA) [ 130 ]. China, Japan, Russia, Singapore, and Malaysia exhibit a more selective regulatory framework. China and Russia allow the online sale of OTC medicines only, with China imposing additional restrictions on third-party e-commerce platforms and Russia having introduced a draft law in December 2017 to formalize this practice [ 131 ; 132 ]. Japan permits the online sale of certain OTC medicines, explicitly excluding specific substances such as fexofenadine and loratadine [ 133 ]. Similarly, Singapore and Malaysia endorse the online sale of specific OTC medicines only, adopting a “buyers beware” approach to caution consumers about the associated risks [ 134 ; 135 ]. Lastly, the legal landscapes in India and Indonesia remain ambiguous. India’s RA has effectively banned the online sale of medicinal products, yet this prohibition lacks legislative backing. Indonesia, too, grapples with unclear regulations, leaving the legal status of online pharmacies indeterminate [ 136 ].
In response to these risks, several initiatives have been developed to guide and certify online pharmacies. In the United States, LegitScript offers certification to online pharmacies that comply with criteria such as appropriate licensing and registration [ 137 ]. Similarly, the Verified Internet Pharmacy Practice Sites (VIPPS) program, accredited by the National Association of Boards of Pharmacy, ensures pharmacies adhere to licensing requirements in the states where they dispense medications [ 138 ]. Internationally, the Health On the Net Foundation has introduced the HONcode, an ethical standard for health websites globally. This code certifies sites that provide transparent and qualified information. However, due to the absence of international harmonization, the HONcode’s certification is limited to US and Canadian pharmacies verified by VIPPS [ 139 ]. The lack of a harmonized international approach presents significant challenges. Consumers do not have access to a comprehensive, global repository of all certified pharmacies. The diverse certification schemes are not well articulated or interconnected, leading to consumer unawareness about their significance or existence. Moreover, enforcing standards across different legal jurisdictions is complex without a unified agreement. To enhance consumer protection, it is imperative to develop and promote a standardized, minimal international code of conduct for online pharmacies. Such a code would unify requirements and allow all initiatives to clarify their roles under a common framework. Adequate oversight in the borderless online pharmacy market can only be achieved through collaborative efforts. To visualize the infographic of the global regularity landscape for the online pharmacy see Fig. 6 .
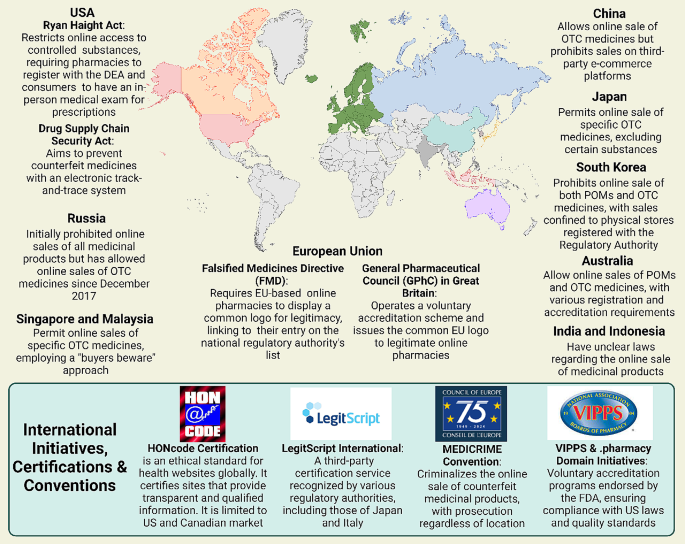
Comprehensive representation of the regulatory landscape for global online pharmacies, detailing international and national initiatives, certification programs, and conventions aimed at minimizing risks associated with the purchase of medications via online platforms
Technological innovations and Future trends in global pharmacy
The global pharmacy sector is undergoing a transformative shift, driven by the rapid advancement of technological innovations. As the world becomes increasingly digital, the integration of cutting-edge technologies like Artificial Intelligence (AI) and blockchain is setting the stage for a new era in pharmaceutical care and management. These advancements promise to revolutionize the industry by enhancing efficiency, accuracy, and security, ultimately leading to improved patient outcomes and a more streamlined healthcare experience [ 140 ].
Walgreens, in partnership with Medline, a telehealth firm, has developed a platform for patient interaction with healthcare professionals via video chat. AI’s role extends to inventory management in retail pharmacies, allowing pharmacists to predict patient needs, stock appropriately, and use personalized software for patient reminders. Although not all inventory management software in retail pharmacies utilizes AI, some, like Blue Yonder’s software developed for Otto group, demonstrate the potential of AI in predicting product sales with high accuracy, thus enhancing supply chain efficiency [ 141 ; 142 ]. At the University of California San Francisco (UCSF) Medical Center, robotic technology is employed to improve patient safety in medication preparation and tracking. This technology has prepared medication doses with a notable error-free record and surpasses human capabilities in accuracy and efficiency. It prepares both oral and injectable medicines, including chemotherapy drugs, freeing pharmacists and nurses to focus on direct patient care. The automated system at UCSF receives electronic medication orders, with robotics handling the picking, packaging, and dispensing of individual doses. This system also assembles medications on bar-coded rings for 12-hour patient intervals and prepares sterile preparations for chemotherapy and intravascular syringes [ 143 ].
In the realm of global pharmacy, blockchain technology emerges as a pivotal force, driving advancements across various facets of healthcare and pharmaceuticals. At the forefront of its application is the enhancement of supply chain transparency [ 144 ]. Blockchain’s immutable ledger ensures the provenance and legitimacy of medical commodities, offering an unprecedented level of visibility from manufacturing to distribution. This is particularly vital in areas plagued by counterfeit drugs, where systems like MediLedger are instrumental in verifying the legality and essential details of medicines [ 145 ].
The utility of blockchain extends to the implementation of smart contracts — scripts processed on the blockchain that bolster transparency in medical studies and secure patient data management [ 146 ]. These contracts find extensive use in advanced medical settings, as evidenced by a blockchain-based telemonitoring system for remote patients and Dermonet, an online platform for dermatological consultation [ 147 ].
Furthermore, blockchain is revolutionizing patient care through patient-centric Electronic Health Records (EHRs). By decentralizing EHR maintenance, blockchain empowers patients with secure access to their historical and current health records [ 148 ]. Prototypes like MedRec and systems such as MeD Share exemplify how blockchain can provide complete, permanent access to clinical documents and facilitate the sharing of medical data between untrusted parties, respectively, ensuring high information authenticity and minimal privacy risks [ 149 ; 150 ]. In verifying medical staff credentials, blockchain again proves invaluable. Systems like ProCredEx, based on the R3 Corda blockchain protocol, streamline the credentialing process, offering rapid verification while allowing healthcare entities to leverage their existing data for enhanced transparency and assurance about medical staff experience [ 151 ].
The integration of blockchain with Internet of Things devices for remote monitoring marks another leap forward, significantly bolstering data security. By safeguarding the integrity and privacy of patient data collected by these devices, blockchain mitigates the risk of tampering and ensures that only authorized parties can access sensitive information [ 152 ]. Besides, a blockchain-based drug supply chain initiative, PharmaChain, utilizes AI for approaches against drug counterfeit and ensures the drug supply chain is more traceable, visible, and secure. For online pharmacies, this means a more reliable supply chain and assurance of drug authenticity, crucial for maintaining trust and safety [ 153 ].
In response to the COVID-19 pandemic, the PharmaGo platform has emerged as an innovative solution in Sri Lanka, revolutionizing the delivery of pharmacy services. As traditional pharmacies grapple with the challenges of meeting all customer needs in one location, PharmaGo addresses this by providing a comprehensive online pharmaceutical service. It allows customers to access a wide range of medications through a single platform, reducing the need to visit multiple pharmacies. Utilizing image processing technology, pharmacy owners can accurately identify prescribed medicines, while the system’s predictive analytics forecasts future drug demands, enhancing stock management. Additionally, PharmaGo’s AI-powered medical chatbot offers real-time guidance, ensuring a seamless and efficient customer experience. This platform represents a significant advancement in healthcare accessibility and pharmacy service delivery in the pandemic era [ 154 ]. In the same context, ontology-based medicine information system, enhancing search relevance through a chatbot interface was presented by Amalia et al. [ 155 ]. Addressing conventional search engines’ limitations in interpreting data relationships, it employs semantic technology to represent metadata informatively. The ontology as a knowledge base effectively delineates disease-medicine relationships, with evaluations indicating a 90% response validity from the chatbot, offering a robust reference for medical information retrieval and its semantic associations.
Future trends for the digital transformation of in the pharmaceutical sector
Future trends for the digital transformation of pharmacies globally are heavily influenced by the transformative impact of digital technologies on healthcare delivery. The integration of telemedicine, electronic health records, and mobile health applications is pivotal in enhancing patient care. These technologies are instrumental in improving data sharing and collaboration among healthcare professionals, increasing the efficiency of healthcare services. Additionally, they offer significant potential for personalized medicine through data analytics and play a crucial role in patient engagement and self-management of health. The importance of these technologies in creating a more connected and efficient healthcare system is underscored, marking a significant shift in the global healthcare landscape [ 156 ].
In the pharmaceutical sector, the COVID-19 pandemic has catalyzed a significant shift towards Pharmaceutical Digital Marketing (PDM), particularly for over-the-counter drugs. This shift focuses on utilizing online pharmacies and digital platforms for targeted advertising, directly reaching consumers. The trend towards purchasing OTC drugs online has grown, driven by the convenience and efficiency of digital channels. While PDM faces challenges like regulatory constraints and the need for digital proficiency, it offers substantial opportunities in enhancing customer engagement and precise marketing. The future of PDM is poised to be more consumer-centric, integrating advanced technologies like AI, and emphasizing personalized marketing strategies to strengthen brand engagement and customer interaction [ 157 ].
Artificial intelligence holds immense potential to revolutionize the field of pharmacy, offering numerous benefits that can significantly enhance efficiency and patient care. One of the primary applications of AI in this sector is the automation of routine tasks. By utilizing AI, pharmacies can automate critical processes such as prescription processing, checking for drug interactions, and managing inventory. This automation not only streamlines operations but also minimizes the likelihood of human error, thereby increasing the overall efficiency of pharmacies [ 158 ].
Furthermore, AI can play a pivotal role in personalized medication management. This is particularly beneficial for patients with chronic conditions such as diabetes who require careful management of their insulin dosages, as fluctuations in blood sugar levels can lead to serious complications. AI systems can monitor patients continuously, provide timely reminders for medication intake, and dynamically adjust treatment plans based on individual health data. Such personalized management ensures that patients receive optimal care tailored to their specific needs, potentially improving treatment outcomes. Incorporation of AI into electronic health records presents another significant advancement. By integrating AI with EHRs, healthcare providers can access real-time patient data. This integration empowers healthcare professionals to make more informed care decisions, enhancing the quality of patient care. Moreover, it significantly reduces the likelihood of medication errors, a critical concern in healthcare.
Likewise, AI’s capability to analyze extensive patient data is invaluable. It can identify patterns and trends in medication adherence, detect potential drug interactions, and pinpoint adverse drug reactions. These insights are crucial for healthcare professionals and researchers. By understanding these patterns, they can develop more effective medication adherence strategies and support systems, contributing to better patient outcomes and advancing the overall field of pharmaceutical care.
In the expansive realm of chemical space, the pharmaceutical industry faces the continual challenge of identifying new active pharmaceutical ingredients (APIs) for diverse diseases [ 159 ]. High throughput screening (HTS), despite its advancements in recent decades, remains resource-intensive and often yields unsuitable hits for drug development. The failure rate of investigational compounds remains high, with a study citing only a 6.2% success rate for orphan drugs progressing from phase I to market approval [ 160 , 161 ].
Machine learning presents a transformative approach to this challenge. It offers an alternative to manual HTS through in silico methodologies. ML-driven drug discovery boasts several advantages: it operates continuously, surpasses the capacity of manual methods, reduces costs by decreasing the number of physical compounds tested, and early identifies negative characteristics of compounds, such as off-target effects and sex-dependent variability [ 162 ].
A substantial advancement in the realm of machine learning has emerged from major pharmaceutical entities, notably AstraZeneca, in conjunction with research institutions. This progress is evidenced by the development of an innovative algorithm that demonstrates both time efficiency and effectiveness in the sphere of drug discovery. The recent introduction of this algorithm significantly enhances the process of determining binding affinities between investigational compounds and therapeutic targets. It surpasses traditional in silico methods in terms of performance. The application of this algorithm underscores the remarkable potential of machine learning in accelerating the identification and development of novel therapeutic agents [ 163 ].
Moreover, the proficiency of machine learning in managing vast and intricate datasets has rendered it indispensable in research focused on cancer targets, utilizing diverse and extensive datasets. This approach is fundamental in numerous drug discovery initiatives, especially those targeting various forms of cancer. A wide array of ML techniques, ranging from supervised to unsupervised learning, are employed to discern chemical attributes that are indicative of potential therapeutic efficacy against a spectrum of cancer targets. This methodology is crucial in identifying novel compounds that could be effective in cancer treatment, leveraging the rich and complex data available in oncological research [ 164 ].
The digital transformation in the pharmacy sector is significantly reshaping healthcare delivery, driven by the integration of cutting-edge technologies like Artificial Intelligence and blockchain. This transformation is marked by a substantial growth in the digital pharmacy market, with a projected annual growth rate of 14.42%, leading to a market volume of approximately $35.33 billion by 2026.
One major aspect of this transformation is the growing reliance on online pharmacy platforms, largely influenced by the COVID-19 pandemic. Consumer trust in online medication purchases has significantly increased, indicating a shift towards digital healthcare solutions. The adoption of telehealth services, including telepharmacy, has surged, with patient adoption in the United States increasing from 11% in 2019 to 46%. This shift towards digital-first services enhances convenience and access to care but also introduces regulatory challenges, particularly in maintaining patient safety and quality standards in the rapidly evolving online healthcare environment.
The cosmeceuticals market, a segment within online pharmacies, is experiencing robust growth. Cosmeceuticals, which bridge the gap between cosmetics and pharmaceuticals, have become a significant part of the skincare industry. The market, valued at USD 56.78 billion in 2022, is projected to expand to USD 95.75 billion by 2030. This expansion is driven by factors like innovation in natural ingredients and significant penetration of internet, smartphone, and social media applications. Despite the growth, the overall penetration for non-specialty drugs in mail-order and online pharmacies remains low, representing a significant portion of specialty prescription revenues. The evolving landscape of online pharmacies in the cosmeceuticals sector reflects a trend towards more accessible and customizable personal healthcare solutions.
Technological innovations are setting the stage for a new era in pharmaceutical care and management. AI’s role extends to areas like inventory management in retail pharmacies, where it predicts patient needs and enhances supply chain efficiency. Blockchain technology enhances supply chain transparency and legitimizes medical commodities, especially crucial in areas affected by counterfeit drugs. Blockchain also plays a vital role in patient-centric Electronic Health Records and telemonitoring systems. For instance, PharmaGo, an innovative platform developed in response to the pandemic, provides a comprehensive online pharmaceutical service, demonstrating the significant advancements in healthcare accessibility and pharmacy service delivery.
These technological advancements are instrumental in improving data sharing and collaboration among healthcare professionals. They offer significant potential for personalized medicine through data analytics, playing a crucial role in patient engagement and self-management of health. The future trends in the pharmaceutical sector, particularly influenced by the COVID-19 pandemic, indicate a shift towards Pharmaceutical Digital Marketing (PDM) and a more consumer-centric approach. AI’s potential in revolutionizing pharmacy includes automation of routine tasks, personalized medication management, real-time patient data access, and the identification of patterns in medication adherence and potential drug interactions.
Data availability
No datasets were generated or analysed during the current study.
Hole G, Hole AS, McFalone-Shaw I. Digitalization in pharmaceutical industry: what to focus on under the digital implementation process? Int J Pharmaceutics: X. 2021;3:100095.
CAS Google Scholar
Shambhavi. Digital Pharmacy on the rise: transforming the Pharma Sector. Brillio.com; Oct. 2022.
Champagne HA, Leclerc D. O., The road to digital success in pharma [Internet]. https://www.mckinsey.com/industries/life-sciences/our-insights/the-road-to-digital-success-in-pharma# ~:text=Mobile%20communications%2 C%20the%20cloud%2 C%20advanced,media%2 C%20retail%2 C%20and%20banking%20industries, August 1, 2015.
COVID-19 accelerated. digital transformation of the pharma industry by five years: Poll, Available from: http://www.pharmaceutical-technology.com/news/covid-19-accelerated-digital-transformation-of-the-pharma-industry-by-five-years-poll/#:~:text=Digital%20transformation%20in%20the%20pharmaceutical,powerful%20analytics%20than%20ever%20before?cf-view&acf-closed , March 9, 2021.
Raza MA, Aziz S, Noreen M, Saeed A, Anjum I, Ahmed M, Raza SM. Artificial Intelligence (AI) in pharmacy: an overview of innovations. INNOVATIONS Pharm 13 (2022).
Zhang X. Investment analysis based on intrinsic value in the Information Technology and Pharmaceutical sectors for advancements in Digital Healthcare. Finance Econ 1 (2023).
Clark M, Clark T, Bhatti A, Aungst T. The rise of digital health and potential implications for pharmacy practice. J Contemp Pharm Pract. 2017;64:32–40.
Article Google Scholar
Health C, Pharmacy CVS. USA, 2023.
MyChart. Epic’s MyChart software system, USA, 2023.
Medisafe MD, Companion. USA, 2023.
Moodle. Moodle open source learning management system, USA, 2023.
PLUS SG. SimMan 3G PLUS advanced emergency care patient simulators, USA, 2023.
Association AP. American Pharmacists Association, USA, 2023.
Fittler A, Ambrus T, Serefko A, Smejkalová L, Kijewska A, Szopa A, Káplár M. Attitudes and behaviors regarding online pharmacies in the aftermath of COVID-19 pandemic: at the tipping point towards the new normal. Front Pharmacol. 2022;13:1070473.
Article PubMed PubMed Central Google Scholar
Hiskey O. The era of telehealth pharmacy practice. J Am Pharmacists Association. 2022;62:10–1.
Plantado ANR, de Guzman HJd, Mariano JEC, Salvan MRAR, Benosa CAC, Robles YR. Development of an online telepharmacy service in the Philippines and analysis of its usage during the COVID-19 pandemic. J Pharm Pract. 2023;36:227–37.
Article PubMed Google Scholar
Zhang PC. The future of pharmacy is intertwined with digital health innovation. Can Pharmacists Journal/Revue Des Pharmaciens Du Can. 2022;155:7–8.
Miller R, Wafula F, Onoka CA, Saligram P, Musiega A, Ogira D, Okpani I, Ejughemre U, Murthy S, Garimella S. When technology precedes regulation: the challenges and opportunities of e-pharmacy in low-income and middle-income countries. BMJ Global Health 6 (2021).
Shawaqfeh MS, Al Bekairy AM, Al-Azayzih A, Alkatheri AA, Qandil AM, Obaidat AA, Harbi SA, Muflih SM. Pharmacy students perceptions of their distance online learning experience during the COVID-19 pandemic: a cross-sectional survey study. J Med Educ Curric Dev. 2020;7:2382120520963039.
Lee CY, Lee SWH. Impact of the educational technology use in undergraduate pharmacy teaching and learning–A systematic review. Pharm Educ. 2021;21:159–68.
Strawbridge J, Hayden JC, Robson T, Flood M, Cullinan S, Lynch M, Morgan AT, O’Brien F, Reynolds R, Kerrigan SW. Educating pharmacy students through a pandemic: reflecting on our COVID-19 experience. Res Social Administrative Pharm. 2022;18:3204–9.
Lin B, Wu S. Digital transformation in personalized medicine with artificial intelligence and the internet of medical things. OMICS. 2022;26:77–81.
Article CAS PubMed Google Scholar
Company M. Trends disrupting pharmacy value pools and potential implications for the value chain, 2018.
Stoumpos AI, Kitsios F, Talias MA. Digital Transformation in Healthcare: Technology Acceptance and its applications. Int J Environ Res Public Health. 2023;20:3407.
Kraus S, Schiavone F, Pluzhnikova A, Invernizzi AC. Digital transformation in healthcare: analyzing the current state-of-research. J Bus Res. 2021;123:557–67.
Jefferies JG, Bishop S, Hibbert S. Customer boundary work to navigate institutional arrangements around service interactions: exploring the case of telehealth. J Bus Res. 2019;105:420–33.
Patrício L, Teixeira JG, Vink J. A service design approach to healthcare innovation: from decision-making to sense-making and institutional change. AMS Rev. 2019;9:115–20.
Hong KS, Lee D. Impact of operational innovations on customer loyalty in the healthcare sector. Service Bus. 2018;12:575–600.
Laurenza E, Quintano M, Schiavone F, Vrontis D. The effect of digital technologies adoption in healthcare industry: a case based analysis. Bus Process Manage J. 2018;24:1124–44.
Mazor I, Heart T, Even A. Simulating the impact of an online digital dashboard in emergency departments on patients length of stay. J Decis Syst. 2016;25:343–53.
Rubbio I, Bruccoleri M, Pietrosi A, Ragonese B. Digital health technology enhances resilient behaviour: evidence from the ward. Int J Oper Prod Manage. 2020;40:34–67.
Hikmet N, Bhattacherjee A, Menachemi N, Kayhan VO, Brooks RG. The role of organizational factors in the adoption of healthcare information technology in Florida hospitals. Health Care Manag Sci. 2008;11:1–9.
Agarwal R, Gao G, DesRoches C, Jha AK. Research commentary—the digital transformation of healthcare: current status and the road ahead. Inform Syst Res. 2010;21:796–809.
Cucciniello M, Lapsley I, Nasi G. Managing health care in the digital world: a comparative analysis. Health Serv Manage Res. 2016;29:132–42.
Eden R, Burton-Jones A, Casey V, Draheim M. Digital transformation requires workforce transformation. MIS Q Exec. 2019;18:1–17.
Google Scholar
Huber C, Gärtner C. Digital transformations in healthcare professionals’ work: Dynamics of autonomy, control and accountability. Manage Revue. 2018;29:139–61.
Burtch G, Chan J. Investigating the relationship between medical crowdfunding and personal bankruptcy in the United States: evidence of a digital divide. MIS Quarterly (Forthcoming; 2018.
Seddon JJJM, Currie WL. Healthcare financialisation and the digital divide in the European Union: narrative and numbers. Inf Manag. 2017;54:1084–96.
Marques IC, Ferreira JJ. Digital transformation in the area of health: systematic review of 45 years of evolution. Health Technol. 2020;10:575–86.
Naik N, Hameed B, Sooriyaperakasam N, Vinayahalingam S, Patil V, Smriti K, Saxena J, Shah M, Ibrahim S, Singh A. Transforming healthcare through a digital revolution: a review of digital healthcare technologies and solutions. Front Digit Health. 2022;4:919985.
Canada. Virtual care policy framework, Canada, 2023.
Dhaliwall S. Telepharmacy services in Canada. Canada: Hospital News; 2018.
Telehealth HHSgov. Telehealth services in the United States, USA, 2023.
HRSA. Health Resources & Services Administration, USA, 2023.
UK.digital. health, Digital health and care, UK, 2023.
Australia AT, Services. Australia, 2023.
Imenokhoeva M. Telehealth in the European Union: Improving Access to Healthcare, HIMSS, EU, 2020.
Lucy d’Arville, Boulton A, Kapur V. Asia Pacific, Telehealth Adoption is expected to soar through 2024. BIAN and Company; 2022.
Intan Sabrina M, Defi IR. Telemedicine guidelines in South East Asia—a scoping review. Front Neurol. 2021;11:581649.
Wolf.Theiss. Telemedicine– the future of healthcare in Central and Eastern Europe, 2022.
Al-Samarraie H, Ghazal S, Alzahrani AI, Moody L. Telemedicine in Middle Eastern countries: Progress, barriers, and policy recommendations. Int J Med Informatics. 2020;141:104232.
Mahdi AlBasri H, Elwan P, Georgiev, Ustun A. Growth opportunities for digital health in KSA and UAE. McKinsey: McKinsey; 2022.
Ibrahim OM, Ibrahim RM, Al Meslamani AZ, Al Mazrouei N. Role of telepharmacy in pharmacist counselling to coronavirus disease 2019 patients and medication dispensing errors. J Telemed Telecare. 2023;29:18–27.
Unni EJ, Patel K, Beazer IR. Hung, Telepharmacy during COVID-19: a scoping review. Pharmacy. 2021;9:183.
Leila Shafiee Hanjani JS, Bell, Freeman CR. Undertaking medication review by telehealth. Australian J Gen Pract. 2020;49:826–31.
Bejarano AP, Santos PV, Robustillo-Cortés MdlA, Gómez ES, Rubio MDS. Implementation of a novel home delivery service during pandemic. Eur J Hosp Pharm (2020) ejhpharm–2020.
Elson EC, Oermann C, Duehlmeyer S, Bledsoe S. Use of telemedicine to provide clinical pharmacy services during the SARS-CoV-2 pandemic. Am J Health-System Pharm. 2020;77:1005–6.
Li H, Zheng S, Liu F, Liu W, Zhao R. Fighting against COVID-19: innovative strategies for clinical pharmacists. Res Social Administrative Pharm. 2021;17:1813–8.
Margusino-Framiñán L, Illarro-Uranga A, Lorenzo-Lorenzo K, Monte-Boquet E, Márquez-Saavedra E, Fernández-Bargiela N, Gómez-Gómez D, Lago-Rivero N, Poveda-Andrés JL, Díaz-Acedo R, Hurtado-Bouza JL, Sánchez-Gundín J, Casanova-Martínez C. Morillo-Verdugo, Pharmaceutical care to hospital outpatients during the COVID-19 pandemic. Telepharmacy Farmacia Hospitalaria. 2020;44:61–5.
PubMed Google Scholar
Hua X, Gu M, Zeng F, Hu H, Zhou T, Zhang Y, Shi C. Pharmacy administration and pharmaceutical care practice in a module hospital during the COVID-19 epidemic. J Am Pharmacists Association. 2020;60:431–e4381.
Asseri AA, Manna MM, Yasin IM, Moustafa MM, Roubie FM, El-Anssasy SM, Baqawie SK. Implementation and evaluation of telepharmacy during COVID-19 pandemic in an academic medical city in the Kingdom of Saudi Arabia: paving the way for telepharmacy. World J Adv Res Reviews. 2020;7:218–26.
Segal EM, Alwan L, Pitney C, Taketa C, Indorf A, Held L, Lee KS, Son M, Chi M, Diamantides E, Gosser R. Establishing clinical pharmacist telehealth services during the COVID-19 pandemic. Am J Health-System Pharm. 2020;77:1403–8.
Bukhari N, Rasheed H, Nayyer B, Babar Z-U-D. Pharmacists at the frontline beating the COVID-19 pandemic. J Pharm Policy Pract. 2020;13:8.
Smith RF. The private sector is taking the lead on enabling digital inclusion. Here’s how. in: W.E. Forum, editor, World Economic Forum, 2021.
Dal Mas F, Massaro M, Rippa P, Secundo G. The challenges of digital transformation in healthcare: an interdisciplinary literature review, framework, and future research agenda. Technovation. 2023;123:102716.
Ianculescu M, Alexandru A. Microservices–A Catalyzer for Better Managing Healthcare Data Empowerment. Stud Inf Control. 2020;29:231–42.
López-Martínez F, Núñez-Valdez ER, García-Díaz V, Bursac Z. A case study for a big data and machine learning platform to improve medical decision support in population health management. Algorithms. 2020;13:102.
Ganapathy K, Reddy S. Technology enabled remote healthcare in public private partnership mode: A story from India. Telemedicine, Telehealth and Telepresence: Principles, Strategies, Applications, and New Directions (2021) 197–233.
Gleiss A, Kohlhagen M, Pousttchi K. An apple a day–how the platform economy impacts value creation in the healthcare market. Electron Markets. 2021;31:849–76.
Bonacina S, Koch S, Meneses I, Chronaki C. Can the European EHR exchange format support shared decision making and citizen-driven health science? Public Health and Informatics, IOS; 2021. pp. 1056–60.
Samuel L, TRANSFORMING THE HEALTHCARE SYSTEM: THE PUBLIC-PRIVATE HEALTHCARE DICHOTOMY IN INDIA IN THE ERA OF DIGITAL HEALTH., Proceedings of the International Conference on Public Health, 2020, pp. 18–31.
Antarsih NR, Setyawati SP, Ningsih S, Sulaiman E, Pujiastuti N. Telehealth Business Potential in Indonesia, International Conference on Social, Economics, Business, and Education (ICSEBE 2021), Atlantis Press, 2022, pp. 73–78.
Loccioni LG. Italy, 2023.
Casprini E, Palumbo R. Reaping the benefits of digital transformation through Public-Private Partnership: A service ecosystem view applied to healthcare. Global Public Policy Gov. 2022;2:453–76.
APOTECAchemo. APOTECAchemo Advanced Robotic Chemotherapy Drug Compounding Systems, Italy, 2023.
Velayati F, Ayatollahi H, Hemmat M, Dehghan R. Telehealth business models and their components: systematic review. J Med Internet Res. 2022;24:e33128.
Barker GP, Krupinski EA, McNeely RA, Holcomb MJ, Lopez AM, Weinstein RS. The Arizona telemedicine program business model. J Telemed Telecare. 2005;11:397–402.
Lee Y-L, Chang P. Modeling a mobile health management business model for chronic kidney disease, Nursing Informatics 2016, IOS Press, 2016, pp. 1047–1048.
Dijkstra SJ, Jurriëns JA, van der Mei RD. A business model for telemonitoring services, 14th Annual High Technology Small Firms Conference, HTSF 2006, University of Twente, 2006.
Grustam AS, Vrijhoef HJ, Poulikidis V, Koymans R, Severens JL. Extending the business-to-business (B2B) model towards a business-to-consumer (B2C) model for telemonitoring patients with chronic heart failure. J Bus Models. 2018;6:106–29.
Lin T-C, Chang H-J, Huang C-C. An analysis of telemedicine in Taiwan: a business model perspective. Int J Gerontol. 2011;5:189–92.
Peters C, Blohm I, Leimeister JM. Anatomy of successful business models for complex services: insights from the telemedicine field. J Manage Inform Syst. 2015;32:75–104.
Lin S-H, Liu J-H, Wei J, Yin W-H, Chen H-H, Chiu W-T. A business model analysis of telecardiology service. Telemedicine e-Health. 2010;16:1067–73.
Mun SK, Tohme WG, Platenberg RC, Choi I. Teleradiology and emerging business models. J Telemed Telecare. 2005;11:271.
Kijl B, Nieuwenhuis LJ, Veld RM, Hermens HJ. and M.M. Vollenbroek-Hutten, Deployment of e-health services–a business model engineering strategy. Journal of telemedicine and telecare 16 (2010) 344–353.
Fusco F, Turchetti G, Interactive Business Models for Telerehabilitation after Total Knee Replacement.: Preliminary Results from Tuscany, Bioinformatics and Biomedical Engineering: Third International Conference, IWBBIO 2015, Granada, Spain, April 15–17, 2015. Proceedings, Part II 3, Springer, 2015, pp. 502–511.
Hidefjäll P, Titkova D. Business model design for a wearable biofeedback system, pHealth, 2015, pp. 213–224.
Oderanti FO, Li F. A holistic review and framework for sustainable business models for assisted living technologies and services. Int J Healthc Technol Manage. 2016;15:273–307.
Valbuena VS, Seelye S, Sjoding MW, Valley TS, Dickson RP, Gay SE, Claar D, Prescott HC, Iwashyna TJ. Racial bias and reproducibility in pulse oximetry among medical and surgical inpatients in general care in the Veterans Health Administration 2013-19: multicenter, retrospective cohort study. BMJ 378 (2022).
Bai G, Rajgopal S, Srivastava A, Zhao R. Profitability and risk-return comparison across health care industries, evidence from publicly traded companies 2010–2019. PLoS ONE. 2022;17:e0275245.
Article CAS PubMed PubMed Central Google Scholar
McCall CJ, DeCaprio D, Gartner J. The Measurement and Mitigation of Algorithmic Bias and Unfairness in Healthcare AI Models Developed for the CMS AI Health Outcomes Challenge. medRxiv (2022) 2022.09. 29.22280537.
Hsueh P-YS. Ecosystem of patient-centered research and information System Design, Personal Health Informatics: patient participation in Precision Health. Springer; 2022. pp. 329–51.
Martin KI, Glaser DA. Cosmeceuticals: the new medicine of beauty. Mo Med. 2011;108:60.
PubMed PubMed Central Google Scholar
Pandey A, Jatana GK, Sonthalia S, Cosmeceuticals SP. StatPearls Publishing Copyright © 2023, StatPearls Publishing LLC., Treasure Island (FL) ineligible companies. Disclosure: Gurpoonam Jatana declares no relevant financial relationships with ineligible companies. Disclosure: Sidharth Sonthalia declares no relevant financial relationships with ineligible companies., 2023.
Morganti P, Morganti G, Gagliardini A, Lohani A. From cosmetics to innovative cosmeceuticals—Non-woven tissues as New Biodegradable Carriers. Cosmetics. 2021;8:65.
Article CAS Google Scholar
Market C. Fortune Business Insights., Cosmeceuticals Market, 2023.
LLP SMR. Global Cosmeceuticals Market, A $95.75 Billion Industry Opportunity by 2030., 2022.
R.a. Markets, Global Cosmeceuticals Market - Outlook & Forecast 2023–2028, 2023.
Research SM. Industry Report and Statistics (Facts & Figures) - Number of Users, Price, Demand & Sales Analysis by Product & Distribution Channel, 2021.
Company M. Meeting changing consumer needs: The US retail pharmacy of the future, 2023.
Ascenso FNS. L’Oréal Portugal: a digital challenge for the active cosmetics division, 2016.
cosmeticaactiva. L’Oreal E-Learning Platform in Portugal, 2023.
Bach D, Newman AL. Governing lipitor and lipstick: capacity, sequencing, and power in international pharmaceutical and cosmetics regulation. Rev Int Polit Econ. 2010;17:665–95.
Organization WH. Quality assurance of pharmaceuticals. a compendium of guidelines and related materials: Good manufacturing practices and inspection, 2007.
Rai S, Gupta A, Punetha V. Regulations of Cosmetics across the Globe. Applied Clinical Research. Clin Trials Regul Affairs. 2015;2:137–44.
Natural VLAMBERT. & Organic Living; Say ‘No’ To Chemicals For A Healthy Life. in: Raconteur, editor, 2016.
Agency EM. The European regulatory system for medicines, 2023.
FDA, Food and Drug Adimenstration in the United States of America, 2023.
Cosmetics I, Market. Industry, Entrepreneurship and SMEs, EU, European Commission 2023.
EEU EEU. TECHNICAL REGULATION ON THE SAFETY OF COSMETICS AND PERFUMERY, 2015.
Danghyan L. Armenian regulatory framework of the cosmetics and pharmaceutical industries, and its compliance with international regulations. Whether the existing regulatory framework of cosmetics and pharmaceutical industries complies with international best practice and ensures product safety and consumer protection. American University of Armenia; 2020.
TGA. Australian Public Assessment Report for Bimatoprost, 2017.
Kwa M, Welty LJ, Xu S. Adverse events reported to the US Food and Drug Administration for cosmetics and personal care products. JAMA Intern Med. 2017;177:1202–4.
DeKoven JG, Warshaw EM, Belsito DV, Sasseville D, Maibach HI, Taylor JS, Marks JG, Fowler JF, Mathias CT, DeLeo VA. North American contact dermatitis group patch test results 2013–2014. Dermatitis. 2017;28:33–46.
Janetos TM, Kwa M, Xu S. Regulation of cosmetics. JAMA Intern Med. 2018;178:1000–1.
Daum C. Self-Regulation in the Cosmetic Industry: A Necessary Reality or a Cosmetic Illusion? 2006.
Schaffer S, Reading Our Lips.: The History of Lipstick Regulation in Western Seats of Power, 2006.
Lincoln JE. Overview of the us fda gmps: good manufacturing practice (gmp)/quality system (qs) regulation (21 CFR part 820). J Validation Technol. 2012;18:17.
van der Heijden I, Pletneva N, Boyer C. How to protect consumers against the risks posed by the online pharmacy market. Swiss Med Inf 29 (2013).
Agency EM. Buying medicines online, 2015.
Union E. Buying medicine online, 2023.
ISPE. Regulating Online Pharmacies & Medicinal Product E-Commerce, 2019.
Swissmedic. Guideline on medicines and the Internet, 2020.
Kulali H. Online Pharmacy in Switzerland: A Full Guide. 2023, Pharmapidya, 2023.
Swissmedic. Current law, 2019.
El Hajj MS, Mekkawi R, Elkaffash R, Saleh R, Awaisi AE, Wilbur K. Public attitudes towards community pharmacy in Arabic speaking Middle Eastern countries: a systematic review. Res Social Administrative Pharm. 2021;17:1373–95.
DelveInsight. The Regulatory process for drug approval in the MENA region, DelveInsight, 2019.
Sallom H, Abdi A, Halboup AM, Başgut B. Evaluation of pharmaceutical care services in the Middle East Countries: a review of studies of 2013–2020. BMC Public Health. 2023;23:1364.
P.B.o. Australia, Guidelines for Dispensing of Medicines., 2019.
Center KLT. Statutes of the Republic of Korea. Pharmaceutical Affairs Act; 2016.
Goryachev I. Russia Federation: Telemedicine Law in Russia, Mondaq, 2018.
Jing M. China FDA stops online med sales, 2016.
L.a.W. o.J. Ministry of Health, List of Sales Sites for over-the-counter Drugs, 2020.
H.S.A.o, Singapore. Dangers of Buying Health Products Online, 2023.
M.o.H.o. Malaysia, Buying Medicines Online: Beware., 2023.
Ganapathy N. India’s pharmacies do battle with online rivals Straitimes, India, 2017.
LegitScript LS. Industry-Leading Certification, 2023.
NABP. The Verified Internet Pharmacy Practice Sites, 2012.
Boyer C, Baujard V, Geissbuhler A. Evolution of health web certification through the HONcode experience. Stud Health Technol Inf. 2011;169:53–7.
Tagde P, Tagde S, Bhattacharya T, Tagde P, Chopra H, Akter R, Kaushik D, Rahman MH. Blockchain and artificial intelligence technology in e-Health. Environ Sci Pollut Res. 2021;28:52810–31.
Walgreen. Convenient virtual care., 2023.
Group O. Otto Group, 2023.
U.o.C.S, Francisco, New UCSF. Robotic Pharmacy Aims to Improve Patient Safety, 2011.
Narayanaswami C, Nooyi R, Govindaswamy SR, Viswanathan R. Blockchain anchored supply chain automation. IBM J Res Dev. 2019;63:7: 1–7.
Khezr S, Moniruzzaman M, Yassine A, Benlamri R. Blockchain technology in healthcare: a comprehensive review and directions for future research. Appl Sci. 2019;9:1736.
Xia Q, Sifah EB, Asamoah KO, Gao J, Du X, Guizani M. MeDShare: Trust-less medical data sharing among cloud service providers via blockchain. IEEE access 5 (2017) 14757–14767.
Mannaro K, Pinna A, Marchesi M. Crypto-trading: Blockchain-oriented energy market, 2017 AEIT International Annual Conference, IEEE, 2017, pp. 1–5.
Rallapalli S, Minalkar A. Improving Healthcare-Big Data Analytics for. J Adv Inform Technol 7 (2016).
Wang H, Song Y. Secure cloud-based EHR system using attribute-based cryptosystem and blockchain. J Med Syst. 2018;42:152.
Cyran MA. Blockchain as a foundation for sharing healthcare data. Blockchain Healthc Today (2018).
Funk E, Riddell J, Ankel F, Cabrera D. Blockchain technology: a data framework to improve validity, trust, and accountability of information exchange in health professions education. Acad Med. 2018;93:1791–4.
Khan PW, Byun Y. A blockchain-based secure image encryption scheme for the industrial internet of things. Entropy. 2020;22:175.
Gomasta SS, Dhali A, Tahlil T, Anwar MM, Ali ABMS. PharmaChain: Blockchain-based drug supply chain provenance verification system. Heliyon. 2023;9:e17957.
Gamage RG, Bandara NS, Diyamullage DD, Senadeera KU, Abeywardena KY, Amarasena N. PharmaGo-An Online Pharmaceutical Ordering Platform, 2021 3rd International Conference on Advancements in Computing (ICAC), IEEE, 2021, pp. 365–370.
Amalia A, Sipahutar PYC, Elviwani E, Purnamasari F. Chatbot Implementation with Semantic Technology for Drugs Information Searching System. Journal of Physics: Conference Series 1566 (2020) 012077.
Trenfield SJ, Awad A, McCoubrey LE, Elbadawi M, Goyanes A, Gaisford S, Basit AW. Advancing pharmacy and healthcare with virtual digital technologies. Adv Drug Deliv Rev. 2022;182:114098.
Anis MS, Hassali MA. Pharmaceutical marketing of over-the-counter drugs in the current digital era: a review. Pharm Sci Asia 49 (2022).
Khan O, Parvez M, Kumari P, Parvez S, Ahmad S. The future of pharmacy: how AI is revolutionizing the industry. Intell Pharm. 2023;1:32–40.
Moshawih S, Hadikhani P, Fatima A, Goh HP, Kifli N, Kotra V, Goh KW, Ming LC. Comparative analysis of an anthraquinone and chalcone derivatives-based virtual combinatorial library. A cheminformatics proof-of-concept study. J Mol Graph Model. 2022;117:108307.
Moshawih S, Goh HP, Kifli N, Idris AC, Yassin H, Kotra V, Goh KW, Liew KB, Ming LC. Synergy between machine learning and Natural products Cheminformatics: application to the lead Discovery of anthraquinone derivatives. Chemical Biology & Drug Design; 2022.
Wong CH, Siah KW, Lo AW. Estimation of clinical trial success rates and related parameters. Biostatistics. 2019;20:273–86.
Moshawih S, Goh HP, Kifli N, Darwesh MAE, Ardianto C, Goh KW, Ming LC. Identification and optimization of TDP1 inhibitors from anthraquinone and chalcone derivatives: consensus scoring virtual screening and molecular simulations. J Biomol Struct Dynamics (2023) 1–25.
Moore JH, Margreitter C, Janet JP, Engkvist O, de Groot BL, Gapsys V. Automated relative binding free energy calculations from SMILES to ∆∆G. Commun Chem. 2023;6:82.
Moshawih S, Lim AF, Ardianto C, Goh KW, Kifli N, Goh HP, Jarrar Q, Ming LC. Target-based small Molecule Drug Discovery for Colorectal Cancer: a review of Molecular pathways and in Silico studies. Biomolecules. 2022;12:878.
Download references
Acknowledgements
The researcher would like to thank the Deanship of Scientific Research, Qassim University for funding the publication of this project.
Deanship of Scientific Research, Qassim University
Author information
Authors and affiliations.
Department of Pharmacology, College of Medicine, Qassim University, Buraydah, Saudi Arabia
Ahmad Almeman
You can also search for this author in PubMed Google Scholar
Contributions
Conceived the conceptual framework, Wrote the paper, Designed search strategies, Critically reviewed the manuscript for important intellectual content: A.A.
Corresponding author
Correspondence to Ahmad Almeman .
Ethics declarations
Ethical approval and consent to participate.
Not applicable.
Consent for publication
Competing interests.
The author declares no competing interest.
Additional information
Publisher’s note.
Springer Nature remains neutral with regard to jurisdictional claims in published maps and institutional affiliations.
Rights and permissions
Open Access This article is licensed under a Creative Commons Attribution 4.0 International License, which permits use, sharing, adaptation, distribution and reproduction in any medium or format, as long as you give appropriate credit to the original author(s) and the source, provide a link to the Creative Commons licence, and indicate if changes were made. The images or other third party material in this article are included in the article’s Creative Commons licence, unless indicated otherwise in a credit line to the material. If material is not included in the article’s Creative Commons licence and your intended use is not permitted by statutory regulation or exceeds the permitted use, you will need to obtain permission directly from the copyright holder. To view a copy of this licence, visit http://creativecommons.org/licenses/by/4.0/ . The Creative Commons Public Domain Dedication waiver ( http://creativecommons.org/publicdomain/zero/1.0/ ) applies to the data made available in this article, unless otherwise stated in a credit line to the data.
Reprints and permissions
About this article
Cite this article.
Almeman, A. The digital transformation in pharmacy: embracing online platforms and the cosmeceutical paradigm shift. J Health Popul Nutr 43 , 60 (2024). https://doi.org/10.1186/s41043-024-00550-2
Download citation
Received : 14 February 2024
Accepted : 09 April 2024
Published : 08 May 2024
DOI : https://doi.org/10.1186/s41043-024-00550-2
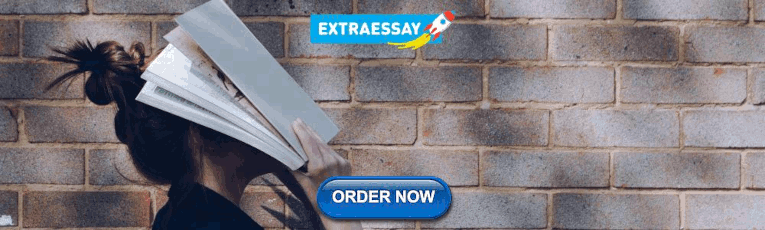
Share this article
Anyone you share the following link with will be able to read this content:
Sorry, a shareable link is not currently available for this article.
Provided by the Springer Nature SharedIt content-sharing initiative
- Digital transformation
- Healthcare technology
- Cosmeceuticals
- Global health
- Patient care
- 2019 novel coronavirus
- Drug safety
Journal of Health, Population and Nutrition
ISSN: 2072-1315
- Submission enquiries: [email protected]
- General enquiries: [email protected]
Pharmaceutical Industry
By Jack McCarthy
Philadelphia played a key role in the birth of the American pharmaceutical industry in the early nineteenth century, and the region remained a major pharmaceutical center into the early twenty-first century. Home since the colonial period to many of America’s leading scientific, educational, and medical institutions, Philadelphia was well-positioned to support the emergence of a pharmaceutical industry. The nation’s first drug mill was established in Philadelphia in the 1810s, and the city hosted a significant concentration of drug makers over the years, as well as related industries such as chemical manufacturing and supporting institutions such as research universities and hospitals.
In the eighteenth and early nineteenth centuries, doctors and pharmacists generally made their own medicines, grinding and mixing materials into powders and extracts themselves. Most medicines were imported from England, however, until the War of 1812 interrupted the supply and spurred domestic drug manufacturing. It was at this point that the American pharmaceutical industry began in earnest. Several Philadelphia pharmacists and chemists branched into small-scale drug manufacturing, and some of these evolved into wholesalers or larger manufacturers.
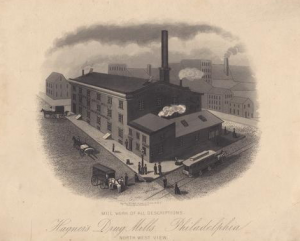
Philadelphia’s first large-scale drug manufacturer was not a pharmacist or chemist, but mill owner Charles Hagner, who in 1812 used his water-powered mill in East Falls to grind several tons of cream of tartar into powder for a local druggist. Hagner’s milling process accomplished in one day what would have taken months by the traditional mortar and pestle method, a feat that generated great interest in the nation’s emerging drug industry. Hagner thereafter focused on grinding materials for medicines, establishing America’s first drug mill at his East Falls location. In 1820 he moved his business to Manayunk , and in 1839 he built a large steam-powered plant in Northern Liberties .
Dozens of Manufacturers Arise
The antebellum period saw the establishment of dozens of pharmaceutical and chemical manufacturers in Philadelphia, fostered by the city’s strong medical, scientific, and educational communities. Powers & Weightman, founded in 1818 by English immigrants, and Rosengarten & Sons, founded in 1822 by a German immigrant, became two of the world’s largest manufacturers of quinine in the nineteenth century. Advertising themselves as “manufacturing chemists,” the two companies made a wide variety of chemicals and medicines before merging in the early twentieth century. John Smith & Company, founded in 1830 by John Smith (d. 1845), and John Wyeth & Brother, founded in 1860 by brothers John (1834–1907) and Frank Wyeth (1836–1913), were two other drug companies established by pharmacists in antebellum Philadelphia. Both began as small-scale operations and grew into pharmaceutical giants. Following various mergers and acquisitions over the years, both remained active in the Philadelphia area in the early twenty-first century.
Robert Shoemaker (1817–96) was yet another early nineteenth-century Philadelphia pharmacist turned drug maker. Shoemaker took over a local pharmacy in 1837 and shortly thereafter began developing glycerin. In 1846 he became the nation’s first commercial manufacturer of the drug. In 1856 William Warner opened a drug store in Philadelphia, where he developed a process for coating medicine tablets with sugar to improve their taste. Warner later gave up his retail business to focus on manufacturing medicines. His company eventually became Warner-Lambert , a major national pharmaceutical firm.
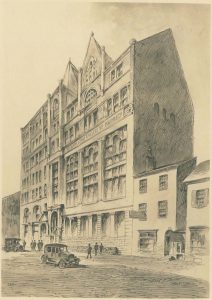
Philadelphia’s status as America’s preeminent early drug manufacturing center was further enhanced by the founding in 1821 of the nation’s first pharmacy college, the Philadelphia College of Pharmacy (PCP), later renamed the University of the Sciences . In addition to training pharmacists, the college’s mission was to “invite a spirit of pharmaceutical investigation and research.” Many of the nation’s leading pharmaceutical executives of the nineteenth and twentieth centuries graduated from PCP.
Philadelphia dominated the American pharmaceutical industry throughout the antebellum period. Forty Philadelphia firms accounted for approximately 30 percent of the total value of the medicines, drugs, and extracts produced in the United States in 1860. Many of these companies benefited significantly from supplying medicines for the Civil War effort and saw considerable growth in the postwar period. In 1872 Wyeth employee Henry Bower (1833–96) developed a rotary tablet machine that allowed for mass production of medicines with precise dosages. The machine greatly expanded Wyeth’s manufacturing capacity and won awards at the 1876 Centennial Exhibition in Philadelphia.
Growth of McNeil and Mulford
Local pharmacists continued to branch into drug manufacturing in the late nineteenth and early twentieth centuries. In 1879 Robert McNeil (1856–1933), a PCP graduate, became proprietor of a drug store in Kensington, from which his son established McNeil Laboratories. The company grew to be one of the region’s major drug makers. Pharmacist Henry Kendall Mulford (1866–1937), also a PCP grad, founded the H. K. Mulford Company in the 1880s from his drug store in downtown Philadelphia. Mulford later focused on making vaccines and in 1895 offered the first commercially available diphtheria antitoxin produced in America. The company built a large plant in Glenolden, Delaware County, which produced several types of vaccines.
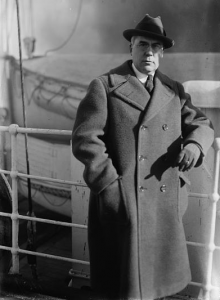
Young chemist Albert Barnes (1872–1951) took a job at H. K. Mulford in 1898, but left the following year to form a partnership with another chemist to make a new antiseptic they developed. After the partnership dissolved in 1908, Barnes created the A. C. Barnes Company to manufacture the product. The company was very successful, and Barnes used the wealth he amassed to collect art and establish the world-renowned Barnes Collection.
Philadelphia was also a major center for the production of “patent medicines,” commercially available over-the-counter nostrums that were sold under brand names. Although their effectiveness was often dubious, patent medicines were marketed with colorful, exaggerated claims of their therapeutic qualities and were very popular in the nineteenth and early twentieth centuries. Thomas Dyott (1771–1861), America’s first successful patent medicine manufacturer, made and sold a variety of elixirs and ointments in Philadelphia in the early nineteenth-century. In the 1810s he built a substantial glass works in Kensington to make bottles for his products. At the turn of the twentieth century, Philadelphia was home to two major patent medicine manufacturers, Dr. D. Jayne & Sons and Johnston, Holloway, & Company.
By 1909, there were 174 drug makers in Philadelphia, along with numerous chemical companies and several major research universities and hospitals. The concentration of these industries and institutions made the region a key center for pharmaceutical research and development throughout the twentieth century. In the early 1940s, West Chester, Pennsylvania, chemist G. Raymond Rettew (1903–73) developed a method for mass producing penicillin, based on his work with mushrooms (mushroom growing was a major industry in Chester County). Sponsored by Wyeth (then operating as American Home Products), Rettew’s Chester County Mushroom Laboratories in West Chester became the world’s leading producer of penicillin , which was put to immediate, extensive use during World War II.
Pharmacy College Influence Persists
Graduates of the Philadelphia College of Pharmacy continued to play leading roles in the local pharmaceutical industry, particularly in family-run companies. At McNeil Laboratories, Robert McNeil, Jr. (1915–2010), grandson of the company founder, joined the firm after graduating from PCP in 1938. In the 1950s he oversaw development of the pain reliever Tylenol, a very successful medicine that became the company’s signature product. Similarly, at William H. Rorer, Inc., founded in 1910, Gerald F. Rorer (1908–76), son of the founder and a 1931 PCP graduate, took over the family-run drug company, based in Fort Washington, Pennsylvania, and transformed it into a major pharmaceutical firm, largely on the strength of its popular antacid, Maalox.
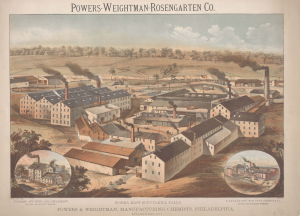
Mergers and acquisitions, common in the pharmaceutical industry from its earliest years, continued over the course of the twentieth century. Powers-Weightman-Rosengarten, formed through the merger of two Philadelphia pharmaceutical giants in 1905, consolidated with Merck & Company in 1927 and moved to northern New Jersey. H. K. Mulford merged with Sharp & Dohme in 1929, which in 1953 was also acquired by Merck & Company . John Smith & Company became Smith, Kline, & French in 1929, and in 2001 merged with English drug maker Glaxo to form GlaxoSmithKline . French multinational firm Rhone-Poulenc acquired Rorer in 1990. Wyeth was acquired in 1932 by American Home Products, parent company to a number of pharmaceutical, home care, and food product manufacturers. American Home Products later divested itself of its non-pharmaceutical companies to focus specifically on drug making and in 2002 adopted the Wyeth name for the entire company. In 2009 Wyeth was acquired by Pfizer, a multinational pharmaceutical company with headquarters in New York City. Nine years earlier, Pfizer had acquired Warner-Lambert.
Increased consolidation among drug makers in the late twentieth and early twenty-first centuries gave rise to “Big Pharma,” a select group of very large multinational pharmaceutical firms that dominated the industry. The Philadelphia area was home to several of these pharmaceutical giants. Some had originally been founded in the city and remained in the region; others moved to the area later in their development. Of the former, the only company still located within the city itself was GlaxoSmithKline, which in 2013 moved one of its two North American headquarters from Center City to the Navy Yard in South Philadelphia. Both Wyeth and McNeil remained in the area but moved to the suburbs, McNeil to Fort Washington, Pennsylvania, in 1961 after being acquired by Johnson & Johnson in 1959, and Wyeth to various suburban locations before consolidating in Collegeville, Pennsylvania, in 2003.
At the turn of the twenty-first century, Philadelphia stood at the center of a mid-Atlantic pharmaceutical corridor stretching from Delaware to northern New Jersey that included many of the world’s Big Pharma companies. In addition to GlaxoSmithKline, Wyeth, and McNeil, the Philadelphia region was home to Endo Pharmaceuticals in Malvern, Pennsylvania, originally established in 1920, later acquired by DuPont, and spun off as a separate company in 1997; AstraZeneca in Wilmington, Delaware, established in 1999 through a merger of two large English and Swedish pharmaceutical companies; and Teva , an Israeli generic drug maker that established a North American headquarters in Montgomeryville, Pennsylvania, in the early 2000s. Numerous other pharmaceutical companies, large and small, were based in the broader Philadelphia metropolitan area in the early twenty-first century as well. They ranged from traditional drug makers to biotechnology firms to research and development companies, all part of the expansive “Life Sciences” sector that comprised a major part of the regional economy. A 2016 report commissioned by a consortium of local business and industry organizations noted that the sector encompassed 1,200 life sciences companies across the region, employed 48,900 workers, and was responsible for $24.6 billion in output.
From its key role in the creation of the American pharmaceutical industry in the early nineteenth century to its position as home to a number of major drug companies in the early twenty-first, the greater Philadelphia region was an important pharmaceutical center for over two hundred years.
Jack McCarthy is an archivist and historian who specializes in three areas of Philadelphia history: music, business and industry, and Northeast Philadelphia. He regularly writes, lectures, and gives tours on these subjects. His book In the Cradle of Industry and Liberty: A History of Manufacturing in Philadelphia was published in 2016 and he curated the 2017–18 exhibit Risk & Reward: Entrepreneurship and the Making of Philadelphia for the Abraham Lincoln Foundation of the Union League of Philadelphia. He serves as consulting archivist for the Philadelphia Orchestra and Mann Music Center and from 2011–16 directed a major archival project for the Historical Society of Pennsylvania focusing on the collections of the region’s many small historical repositories.
Copyright 2018, Rutgers University
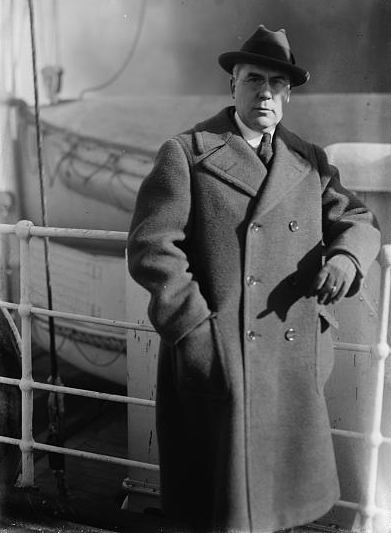
Albert C. Barnes
Library of Congress
Albert C. Barnes (1872–1951) grew up in South Philadelphia and graduated from the University of Pennsylvania School of Medicine in 1892. After studying advanced chemistry in Germany from 1894 to 1895, Barnes returned to Philadelphia, eventually taking a job at the H. K. Mulford pharmaceutical company in 1898.
Barnes left Mulford the following year to manufacture Argyrol, a silver-based antiseptic, with his business partner Hermann Hille (1871–1962). This 1900 photograph was taken around the same time as the product launch. Barnes bought out Hille in 1907 and founded the A. C. Barnes Company in 1908. Over the next two decades, Barnes amassed his fortune and began collecting paintings, many of which remain on view at the Barnes Foundation in Philadelphia.
Barnes sold his company for a significant profit in 1929 and continued collecting art until his death in 1951.
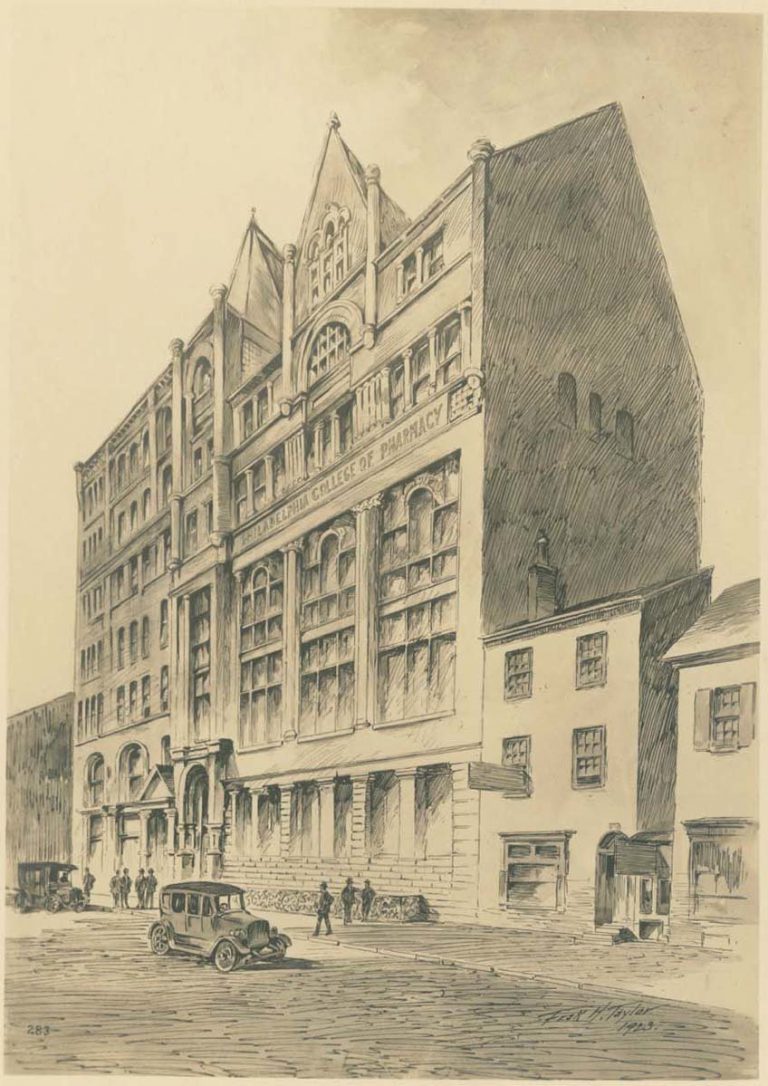
Philadelphia College of Pharmacy
Library Company of Philadelphia
By the early nineteenth century, Philadelphia had emerged as the preeminent drug manufacturing center in the United States. The 1821 founding of the Philadelphia College of Pharmacy further cemented this status.
Several well-known pharmaceutical leaders graduated from PCP, including William R. Warner (1836–1901), John Wyeth (1834–1907), and Robert L. McNeil (1915–2010). William Procter Jr. (1817–1874), one of the co-founders of the American Pharmaceutical Association, served as a faculty member for three decades beginning in 1846.
The college became coeducational in 1876 and later introduced curricula in three additional areas alongside pharmacy: bacteriology, biology, and chemistry. PCP, shown here in a 1922 drawing, was renamed the University of the Sciences in 1998.
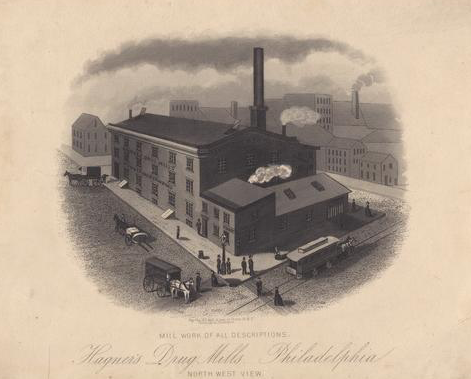
Hagner's Drug Mills
Historical Society of Pennsylvania
Philadelphia’s first large-scale drug manufacturer was not a pharmacist or chemist, but mill owner Charles Hagner (1796–1878). In the eighteenth and early nineteenth centuries, doctors and pharmacists made drugs using the traditional mortar and pestle method. In 1812, Hagner used his water-powered mill in East Falls to grind several tons of cream of tartar into powder for a local druggist, completing in one day a process that would have taken months by the traditional method.
Hagner’s innovation spurred Philadelphia’s emerging drug industry. In the ensuing years, Hagner focused on pharmaceutical production, establishing the first drug mill in the United States at his East Falls location. He moved to Manayunk in 1820 and built a large steam-powered plant in Northern Liberties in 1839. This 1875 lithograph shows one of his mills at Pegg and New Market Streets.
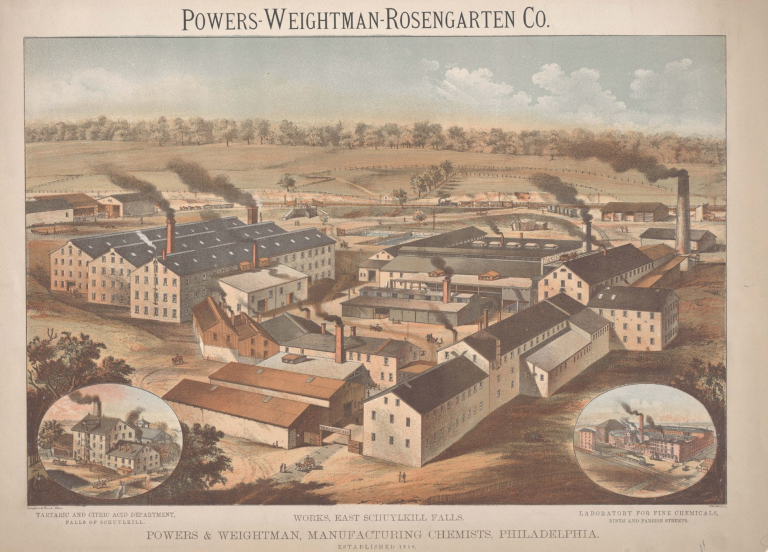
Powers-Weightman-Rosengarten Company
The antebellum period saw the establishment of dozens of pharmaceutical and chemical manufacturers in Philadelphia. Powers & Weightman (est. 1818) and Rosengarten & Sons (est. 1822) became two of the world’s largest manufacturers of quinine, an antimalarial drug.
Both companies made a variety of chemicals and medicines throughout the nineteenth century, and ultimately merged in 1905. This advertisement, produced the same year as the merger, depicts the extensive campus of Powers-Weightman-Rosengarten in East Falls.
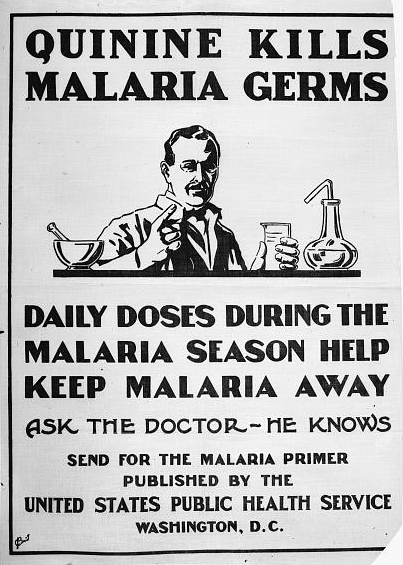
Quinine Poster – U.S. Public Health Service
Quinine, a drug made from cinchona plants, was a common malaria treatment in the nineteenth and early twentieth centuries. Quinine removes malarial parasites from red blood cells and quickly alleviates symptoms of the disease, though malaria can persist in other cells and cause relapse.
Research during World War II led to the discovery of more effective antimalarial drugs, such as chloroquine and primaquine. However, quinine aided many sufferers in the years of its primary use. Several Philadelphia manufacturers, including Powers & Weightman and Rosengarten & Sons, produced quinine for decades. This 1920 poster, released by the U.S. Public Health Service, advertises the benefits of the drug.
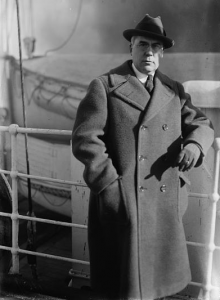
Related Topics
- Workshop of the World
- City of Medicine
- Philadelphia and the World
- Philadelphia and the Nation
- City of Firsts
Time Periods
- Twenty-First Century
- Twentieth Century after 1945
- Twentieth Century to 1945
- Nineteenth Century after 1854
- Nineteenth Century to 1854
- West Philadelphia
- Woman’s Medical College of Pennsylvania
- Chemical Industry
- University City Science Center
- Typhoid Fever and Filtered Water
- Barnes Foundation
- Medical Publishing
- Home Remedies
- Manufacturing Suburbs
Related Reading
England, Joseph W., ed. The First Century of the Philadelphia College of Pharmacy, 1821–1921 . Philadelphia: Philadelphia College of Pharmacy and Science, 1922.
Liebenau, Jonathan. Medical Science and Medical Industry: The Formation of the American Pharmaceutical Industry . London: Macmillan Press, 1987.
Macfarlane, John James. Manufacturing in Philadelphia, 1682–1912 . Philadelphia: Philadelphia Commercial Museum, 1912.
Zebroski, Bob. A Brief History of Pharmacy: Humanities Search for Wellness . New York: Routledge, 2016.
Related Collections
Philadelphia Drug Exchange Records, 1861–1957. Historical Society of Pennsylvania , 1300 Locust Street, Philadelphia.
Related Places
Astra-Zeneca/MedImmune manufacturing facility, 3001 Red Lion Road, Philadelphia.
Endo headquarters, 1400 Atwater Drive, Malvern, Pa.
GlaxoSmithKline headquarters, Philadelphia Navy Yard, 5 Crescent Drive, Philadelphia.
McNeil Consumer Healthcare headquarters, 7050 Camp Hill Road, Fort Washington, Pa.
Rorer and Wyeth headquarters at Pfizer campus, 500 Arcola Road, Collegeville, Pa.
Teva headquarters, 1090 Horsham Road, North Wales, Pa.
University of the Sciences , 600 S. Forty-Third Street, Philadelphia.
- Greater Philadelphia Region Life Sciences Report (PDF, CEO Council for Growth)
- G. Raymond Rettew Historical Marker (ExplorePAHistory.com)
- Philadelphia College of Pharmacy and Science Historical Marker (ExplorePAHistory.com)
- Philadelphia College of Pharmacy (University of the Sciences)
- Quinine - Top Pharmaceuticals (American Chemical Society)
- Sketch of the Interior of the Philadelphia College of Pharmacy (ExplorePAHistory.com)
- The Barnes Foundation
Connecting the Past with the Present, Building Community, Creating a Legacy
- Skip to primary navigation
- Skip to main content
- Skip to primary sidebar
UPSC Coaching, Study Materials, and Mock Exams
Enroll in ClearIAS UPSC Coaching Join Now Log In
Call us: +91-9605741000
Indian Pharmaceutical Industry
Last updated on September 13, 2023 by ClearIAS Team
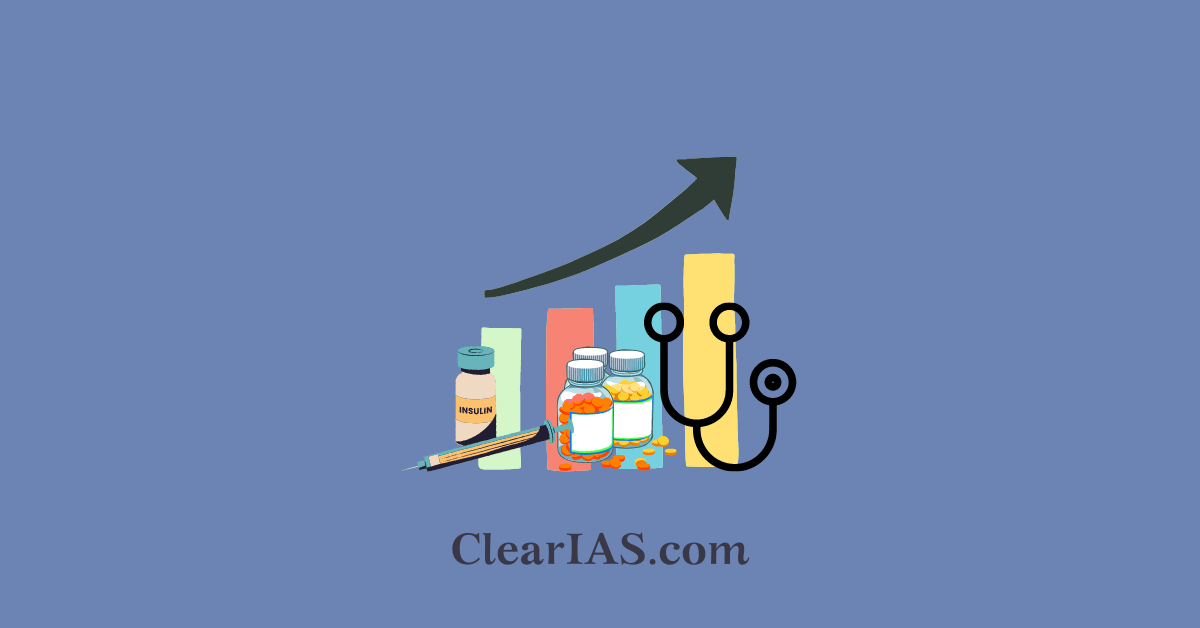
The Indian Pharmaceuticals industry plays a prominent role in the global pharmaceuticals industry. India ranks 3rd worldwide for production by volume and 14th by value. Read here to learn more about the Indian Pharma sector.
India is the largest provider of generic medicines globally, occupying a 20% share in global supply by volume, and is the leading vaccine manufacturer globally.
India also has the highest number of US-FDA-compliant Pharma plants outside of the USA and is home to more than 3,000 pharma companies with a strong network of over 10,500 manufacturing facilities as well as a highly-skilled resource pool.
The pharmaceutical industry in India offers 60,000 generic brands across 60 therapeutic categories. Major segments include generic drugs, OTC Medicines, API/Bulk Drugs, Vaccines, Contract Research & Manufacturing, Biosimilars, and Biologics.
Table of Contents
Indian Pharmaceutical industry
Indian pharma companies enabled by their price competitiveness and good quality, have made a global mark, with 60% of the world’s vaccines and 20% of generic medicines coming from India.
100% Foreign Direct Investment (FDI) in the pharmaceutical sector is allowed under the automatic route for greenfield pharmaceuticals.
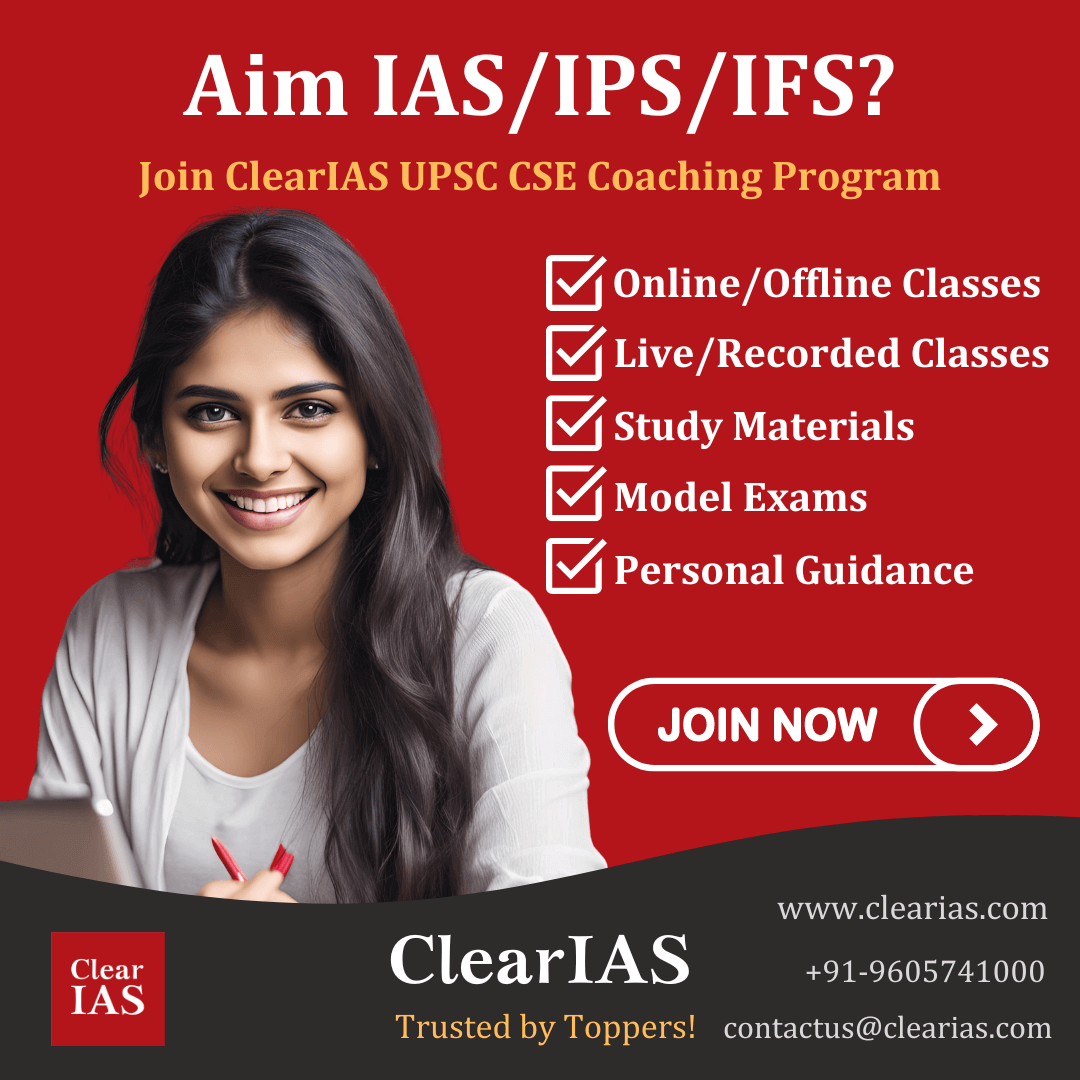
- 100% FDI in the pharmaceutical sector is allowed in brownfield pharmaceuticals; wherein 74% is allowed under the automatic route and thereafter through the government approval route.
India is a major exporter of Pharmaceuticals, with over 200+ countries served by Indian pharma exports.
- India supplies over 50% of Africa’s requirement for generics, ~40% of generic demand in the US, and ~25% of all medicine in the UK.
- India also accounts for ~60% of global vaccine demand and is a leading supplier of DPT, BCG, and Measles vaccines.
- 70% of WHO’s vaccines (as per the essential Immunization schedule) are sourced from India.
The country also has a large pool of scientists and engineers with the potential to steer the industry ahead to greater heights.
- Presently, over 80% of the antiretroviral drugs used globally to combat AIDS (Acquired Immune Deficiency Syndrome) are supplied by Indian pharmaceutical firms.
India is rightfully known as the “pharmacy of the world” due to the low cost and high quality of its medicines.
India is the 12th largest exporter of medical goods in the world.
- Indian drugs are exported to more than 200 countries in the world, with the US being the key market.
- Generic drugs account for 20% of the global export in terms of volume, making the country the largest provider of generic medicines globally.
Advantage India in the Pharmaceutical sector
The main growth drivers of the Indian pharma sector are:
- Government Support: Government incentives like Production linked incentives schemes for the pharma sector.
- Medical tourism: Quality services at marginal costs compared to the US, Europe, and South Asia
- Infrastructure development: India has the highest number of US-FDA-compliant plants outside the US.
- Strong drug manufacturing: Expertise in low-cost generic patented drugs as well as end-to-end manufacturing.
- Strong domestic demand: Launch of the largest National Health Protection Scheme globally.
- Cost efficiency: Low cost of production and R&D boosts the efficiency of Indian pharma companies, leading to competitive exports.
- Economic drivers: High economic growth along with increasing penetration of health insurance to push expenditure on healthcare and medicine in India.
- Increased investment: The FDI inflows in the Indian drugs and pharmaceuticals sector are increasing with each year.
Challenges faced by the Indian pharmaceutical industry
- India has a lot of talent and labor, but its infrastructure for innovation is still lacking. To increase India’s creativity, the government must fund talent and research projects.
- Clinical trials and subjectivity in specific regulatory decision-making should be encouraged by the government.
- India depends substantially on foreign nations for intermediates and active pharmaceutical ingredients (API) . China is the source of imports for 80% of APIs.
- India is thus at the mercy of erratic price changes and supply interruptions. To stabilize supply, it is required to implement infrastructure improvements in the area of internal facilities.
- Since 2009, the Food and Drug Administration (FDA) has conducted the most inspections in India; as a result, continuing investments in raising quality standards would divert money away from other areas of growth and development.
- Due to the difficulty posed by India’s frequent and unforeseen adjustments to its domestic pricing policies; the climate for investments and ideas has become hazy.
Government initiatives for the pharma sector
The Indian pharmaceuticals market is supported by the following Production Linked Incentive Schemes to boost domestic manufacturing capacity, including high-value products across the global supply chain.
- PLI Scheme for Key Starting Materials (KSMs)/Drug Intermediates (DIs) and Active Pharmaceutical Ingredients (APIs) (PLI 1.0)
- Production-Linked Incentive (PLI) Scheme for Pharmaceuticals d (PLI 2.0)
The products under the PLI schemes are divided into 3 categories:
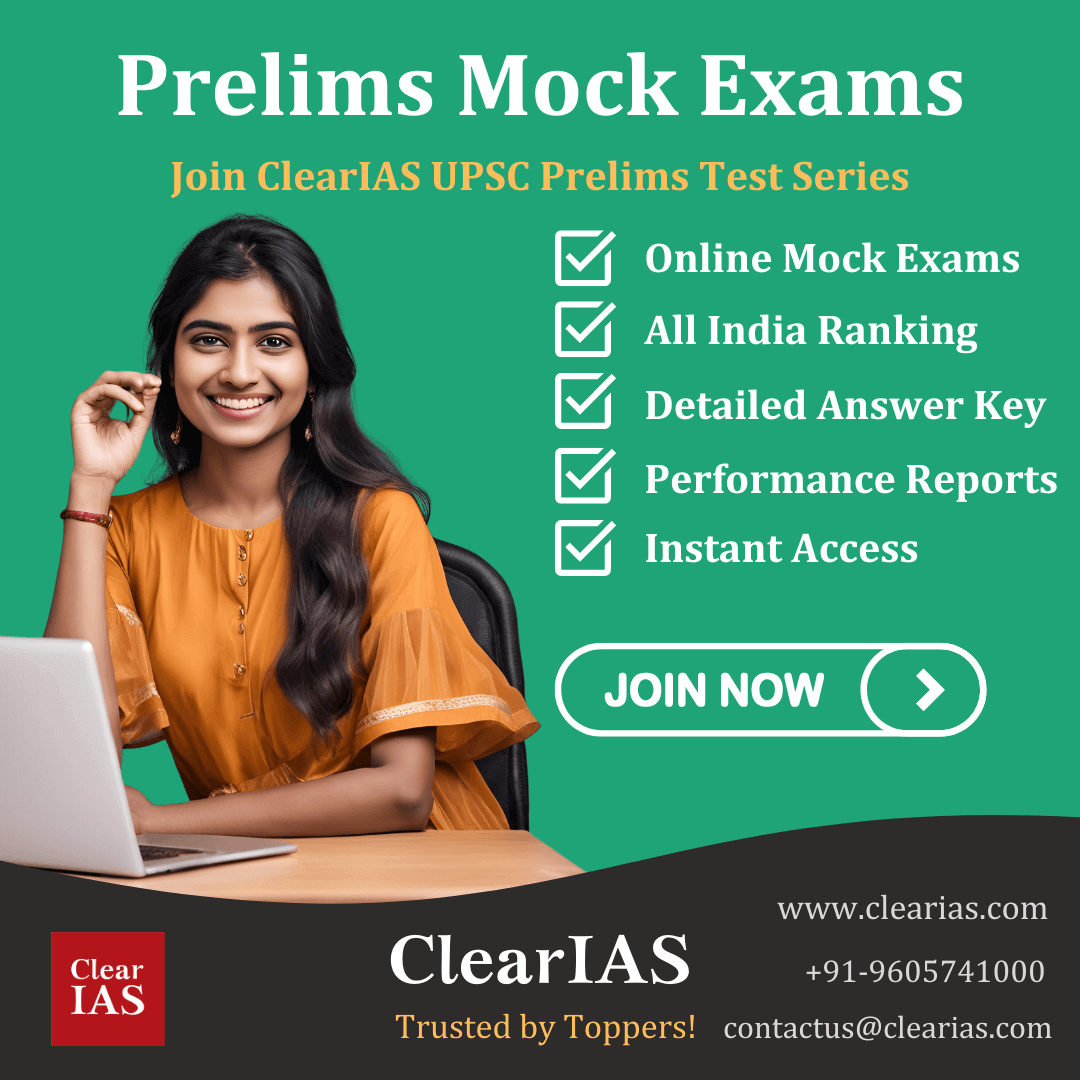
- Category 1: Biopharmaceuticals, Complex generic drugs, Patented drugs or drugs nearing patent expiry, Cell-based or gene therapy products, Orphan drugs, Special empty capsules, Complex excipients, Phyto-pharmaceuticals
- Category 2: Active Pharma Ingredients (APIs), Key Starting Materials (KSMs), Drug Intermediaries (Dls)
- Category 3: Repurposed Drugs, Auto-immune drugs, Anti-cancer drugs, Anti-diabetic drugs, Anti Infective drugs, Cardiovascular drugs, Psychotropic drugs, Anti-Retroviral drugs, Fermentation based 4 KSMs /DIs
Under the ‘ Promotion of Bulk Drug Parks ’ Scheme, the Union government aims to develop 3 mega Bulk Drug parks in India in partnership with the States.
- The parks will have common facilities such as a solvent recovery plant, distillation plant, power & steam units, and a common effluent treatment plant.
Strengthening of Pharmaceutical Industry (SPI) Scheme was announced in 2022 and received a 500-crore financial outlay for the period FY 2021-22 to FY 2025-26.
- The objective of the scheme is to foster resilience and future readiness in the existing infrastructure facilities to make India a global leader in the Pharma Sector.
Promotion of medical devices parks
- The objective of the scheme is the Creation of world-class infrastructure facilities to make the Indian medical device industry a global leader
- Easy access to standard testing and infrastructure facilities through the creation of world-class Common Infrastructure Facilities for increased competitiveness will result in a significant reduction in the cost of production of medical devices leading to better availability and affordability of medical devices in the domestic market.
Scheme for the Establishment of AYUSH Super Specialty Hospitals/ Day Care Centres for Medical Tourism
- The Champion Services Sector Scheme for Medical Value Travel has been framed by Govt. of India to enhance Medical Tourism in the country, especially in the field of the Traditional System of Medicine
- The objective of the scheme is to encourage private investors to invest in the AYUSH sector through the Establishment of World Class, State of Art Super Specialty Hospitals/ Day Care Centres of the systems recognized under the Indian Medicine Central Council (IMCC) Act, 1970 or Homeopathic Central Council (HCC) Act, 1973.
- The scheme is for promoting the export of medical value travel services including services supplied to foreign consumers in India by providing interest subsidy.
Pharmaceuticals Promotion Development Scheme (PPDS)
Under the PPDS, the Department of Pharmaceuticals aims to:
- Conduct Training/knowledge improvement programs/activities on issues/subjects relevant to the growth of the pharmaceutical industry.
- Organize Summits, conventions, Exhibitions, Pharmacy week, meetings, etc. in India and abroad and produce promotional materials like films, displays, etc.
- Conduct research studies, sector reports, etc.
Way forward
The Indian pharmaceutical industry is a significant part of the nation’s foreign trade and offers lucrative potential for investors.
Millions of people around the world receive affordable and inexpensive generic medications from India, which also runs a sizable number of plants that adhere to Good Manufacturing Practices (GMP) standards set by the World Health Organization (WHO) and the United States Food and Drug Administration (USFDA).
The capacity of firms to orient their product portfolio towards chronic treatments for diseases like cardiovascular, anti-diabetes, anti-depressants, and anti-cancers, which are on the increase, will also play a role in future domestic sales growth.
The Indian government has taken several measures to cut expenditures and lower healthcare prices.
- The opening of pharmacies that provide low-cost generic medications as well as other government initiatives, such as the National Health Protection Scheme, which aims to provide universal healthcare, the aging population, the rise in chronic diseases, and other factors should all help to strengthen the Indian pharmaceutical industry.
The quick entry of generic medications into the market has remained a priority and is anticipated to help Indian pharmaceutical businesses. Also, the focus on preventative immunizations, life-saving medications, and rural health programs bodes well for the Indian pharmaceutical industry.
Related posts
- Good Manufacturing Practices (GMP)
- Pradhan Mantri Bhartiya Janaushadhi Pariyojana (PMBJP)
- MSME Competitive (LEAN) Scheme
-Article written by Swathi Satish
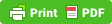
Aim IAS, IPS, or IFS?
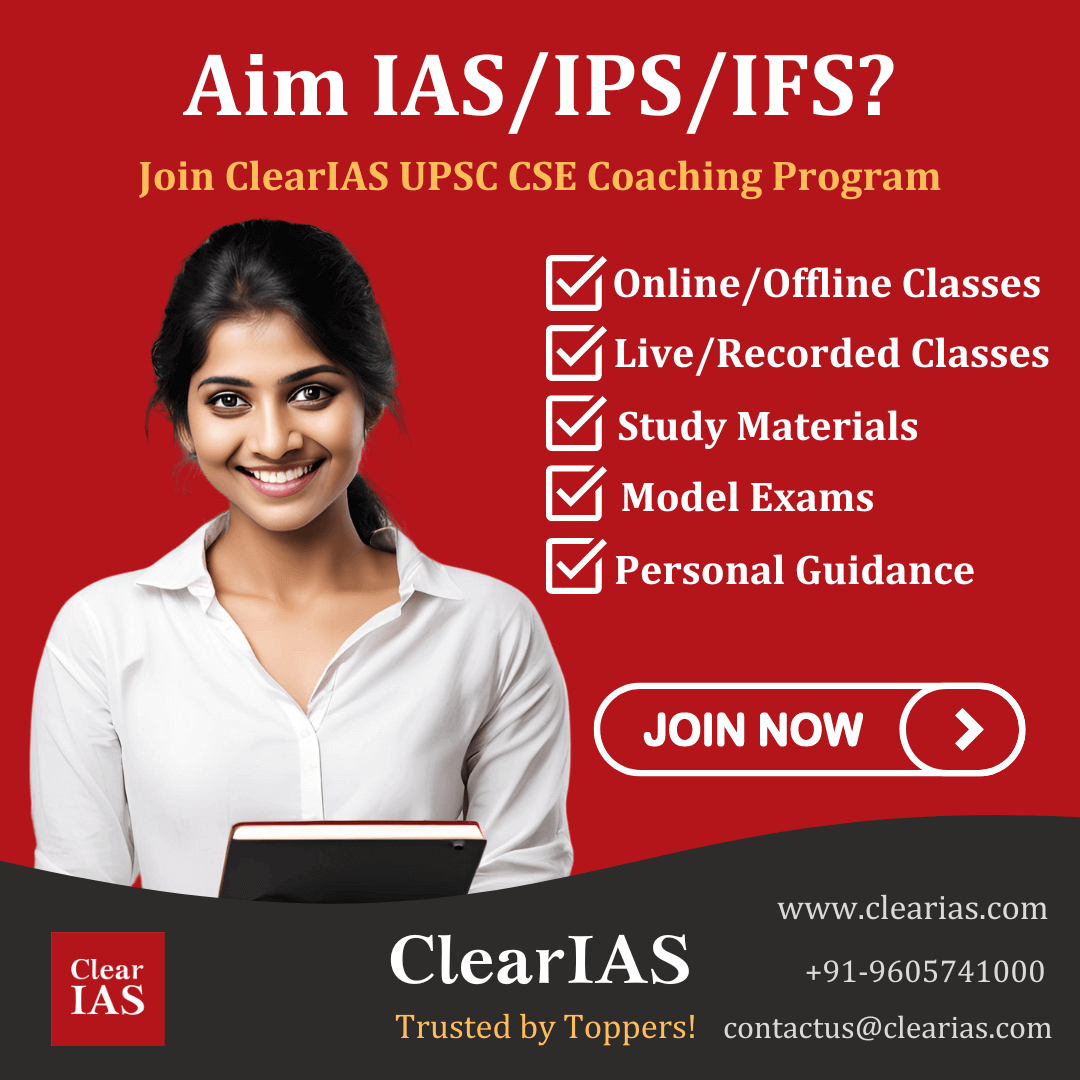
About ClearIAS Team
ClearIAS is one of the most trusted learning platforms in India for UPSC preparation. Around 1 million aspirants learn from the ClearIAS every month.
Our courses and training methods are different from traditional coaching. We give special emphasis on smart work and personal mentorship. Many UPSC toppers thank ClearIAS for our role in their success.
Download the ClearIAS mobile apps now to supplement your self-study efforts with ClearIAS smart-study training.
Reader Interactions
Leave a reply cancel reply.
Your email address will not be published. Required fields are marked *
Don’t lose out without playing the right game!
Follow the ClearIAS Prelims cum Mains (PCM) Integrated Approach.
Join ClearIAS PCM Course Now
UPSC Online Preparation
- Union Public Service Commission (UPSC)
- Indian Administrative Service (IAS)
- Indian Police Service (IPS)
- IAS Exam Eligibility
- UPSC Free Study Materials
- UPSC Exam Guidance
- UPSC Prelims Test Series
- UPSC Syllabus
- UPSC Online
- UPSC Prelims
- UPSC Interview
- UPSC Toppers
- UPSC Previous Year Qns
- UPSC Age Calculator
- UPSC Calendar 2024
- About ClearIAS
- ClearIAS Programs
- ClearIAS Fee Structure
- IAS Coaching
- UPSC Coaching
- UPSC Online Coaching
- ClearIAS Blog
- Important Updates
- Announcements
- Book Review
- ClearIAS App
- Work with us
- Advertise with us
- Privacy Policy
- Terms and Conditions
- Talk to Your Mentor
Featured on
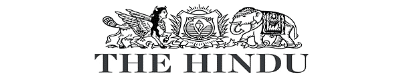
and many more...
Take ClearIAS Mock Exams: Analyse Your Progress
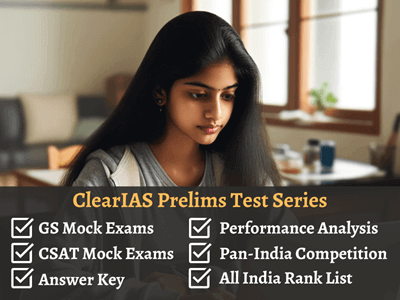
Analyse Your Performance and Track Your All-India Ranking
Ias/ips/ifs online coaching: target cse 2025.
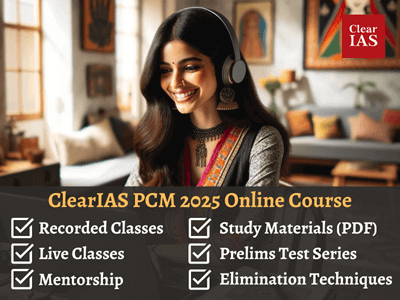
Are you struggling to finish the UPSC CSE syllabus without proper guidance?
- Pharmaceutical Engineering Magazine
- Online Exclusives
- Special Reports
- Facilities & Equipment
- Information Systems
- Product Development
- Production Systems
- Research + Development
- Supply Chain
- White Papers
- iSpeak Blog
- Editorial Calendar
- Article of the Year
- Submit an Article
- Editorial Team
Building Resilient and Sustainable Pharmaceutical Supply Chains
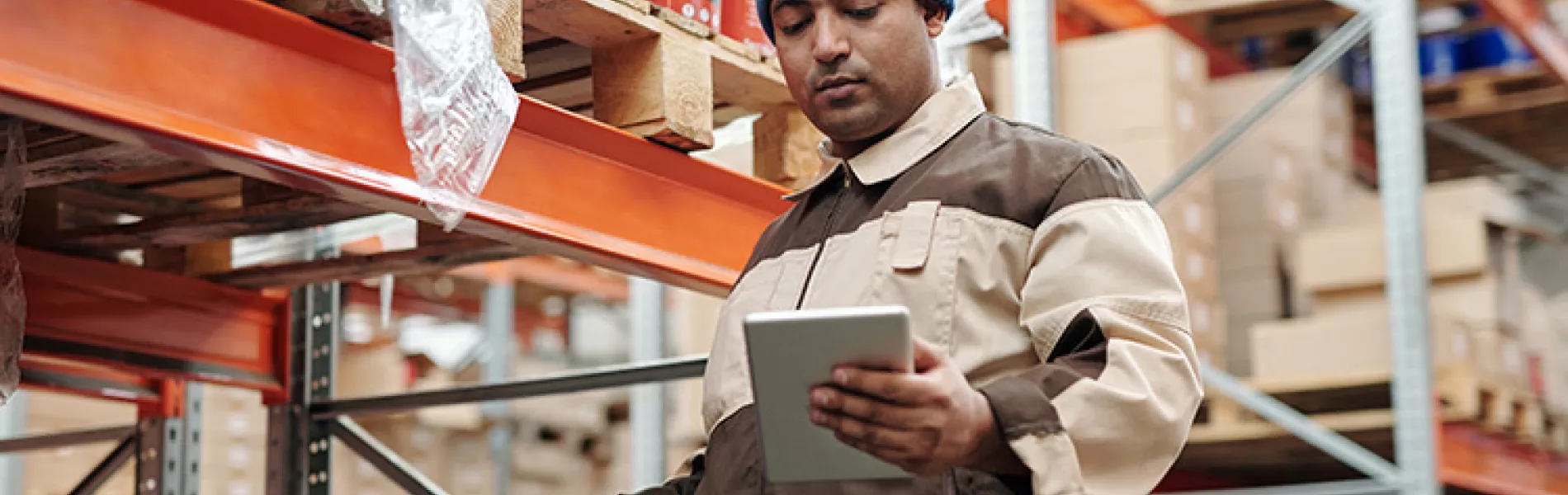
The global pharmaceutical industry has faced unprecedented challenges in recent years, with the COVID-19 pandemic serving as a wake-up call for the vulnerabilities within the pharmaceutical supply chain. As the world strives to emerge from the grip of the pandemic and restore activities reminiscent of pre-pandemic times, uncertainties surrounding supply chain stability persist. The 2023 ISPE Annual Meeting & Expo comes at a crucial time, addressing Supply Chain Resiliency and Sustainability to provide the industry with essential insights, strategies, and best practices.
The conference recognizes that valuable lessons can be learned from the pandemic and applied to future planning for the possibility of another pandemic or even a tripledemic. Global health authorities have been actively developing guidance and recommendations to mitigate disruptions in the supply chain, ensuring secure patient access to critical medicines. Regulatory efforts aim to improve planning and preventative measures, while some governments have implemented legislative measures encouraging onshoring as a protective measure to insulate domestic supply chains from disruption.
In parallel with these efforts, the ISPE Drug Shortages Initiative team has developed expanded guidance on drug shortage prevention, addressing the quality, technical, and regulatory aspects. This initiative demonstrates the industry's proactive approach to secure the supply chain, mitigate drug shortages, and be prepared for the possibility of a future pandemic. The conference will provide a platform for regulators and experts to share their experiences and efforts in securing the supply chain and proactively preparing for future challenges.
With a focus on supply chain resiliency and sustainability, the conference sessions cover a wide range of topics critical to the success of pharmaceutical companies. These include optimizing supply chain operations, lifecycle management, lab transformation, sustainable development, and navigating emerging markets. Attendees can expect to gain valuable insights, practical strategies, and actionable knowledge to thrive in an industry that demands resilience, sustainability, and adaptability.
By coming together at the 2023 ISPE Annual Meeting & Expo, pharmaceutical professionals can collaboratively Shape the Future of Pharma™. With a shared commitment to building resilient and sustainable supply chains, the conference aims to equip attendees with the tools and knowledge needed to navigate uncertainties, mitigate disruptions, and ensure uninterrupted access to life-saving medications for patients worldwide.
Acknowledging Supply Chain Stability Uncertainties:
As the world attempts to emerge from the pandemic and restore pre-pandemic activities, uncertainties surrounding supply chain stability persist. Lessons learned from the pandemic form the foundation for planning and preparing for future crises, including the possibility of another pandemic or even a tripledemic. Regulatory guidance and recommendations from global health authorities have emerged to enhance supply chain resilience and ensure uninterrupted patient access to critical medicines. Additionally, some governments have introduced legislative measures encouraging onshoring to insulate domestic supply chains from disruption. This session emphasizes the importance of aligning with regulatory efforts and offers expanded guidance on drug shortage prevention.
Optimizing Supply Chain Operations and Lifecycle Management:
Pharmaceutical companies face significant challenges in supply chain operations, lifecycle management, and lab transformation. Experts from leading industry players will share their experiences and best practices during this insightful session. They will explore supply chain flexibility and resilience, highlighting strategies that enable multiple pathways for safe and efficient product delivery. Lifecycle management discussions will delve into the challenges posed by supply chain disruption, cost pressures, and regulatory expectations, offering valuable lessons and strategies to maintain continuity of supply and profitability. Additionally, the session will shed light on the impact of digitalization and sustainable practices in lab transformation, focusing on workforce transformation, data integrity, lab quality control, and compliance.
Promoting Sustainable Development and Governance:
Sustainability plays a vital role in building resilient and efficient pharmaceutical supply chains. The conference will feature an engaging session that showcases innovations and best practices in sustainable development and governance. Attendees will gain valuable insights into environmental stewardship, energy efficiency, waste reduction, and responsible manufacturing practices. Integrating sustainability into pharmaceutical operations will be emphasized, highlighting the positive impact it can have on the industry's bottom line and public perception. This session aims to equip attendees with practical strategies to promote sustainability and foster responsible pharmaceutical manufacturing.
Navigating Emerging Markets: Challenges and Opportunities:
Expanding into emerging markets presents unique challenges and opportunities for pharmaceutical companies. A dedicated session will provide valuable insights into the regulatory and market dynamics involved in successfully launching products in these regions. Attendees will gain a comprehensive understanding of the cultural, legal, and operational considerations necessary to navigate emerging markets effectively. Supply chain security and product quality will also be addressed, highlighting the risks, vulnerabilities, and strategies to mitigate them. The session will delve into the role of technology, such as connected labs, in enhancing supply chain security and product quality, ultimately improving patient safety. Finally, The SERUM's NISHWAS project receives the FOYA 2023 award in the "Supply Chain" category for rapidly establishing a pharmaceutical manufacturing capability during a crisis. The Genentech Clinical Supply Center (CSC) project wins the FOYA 2023 Pharma 4.0™ category, showcasing the benefits of digital technologies and innovative planning in improving construction, safety, productivity, and patient access to medicines.
The 2023 ISPE Annual Meeting & Expo offers an exceptional platform for pharmaceutical professionals to gain insights and strategies for building resilient and sustainable supply chains. With a focus on supply chain resiliency and sustainability, attendees will discover practical approaches to navigate uncertainties, mitigate disruptions, and ensure uninterrupted patient access to critical medicines. The conference's sessions will share valuable lessons from the pandemic, best practices in supply chain operations and lifecycle management, and insights into sustainable development and governance. By embracing these insights, the pharmaceutical industry can enhance its resilience, promote sustainability, and continue to meet the healthcare needs of patients worldwide.
We hope you join us in Las Vegas, NV, USA for the 2023 ISPE Annual Meeting & Expo and the Supply Chain Resiliency Sessions!
Learn More & Register
- 'Share on Twitter'
About the Author
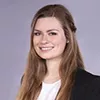
Related Articles
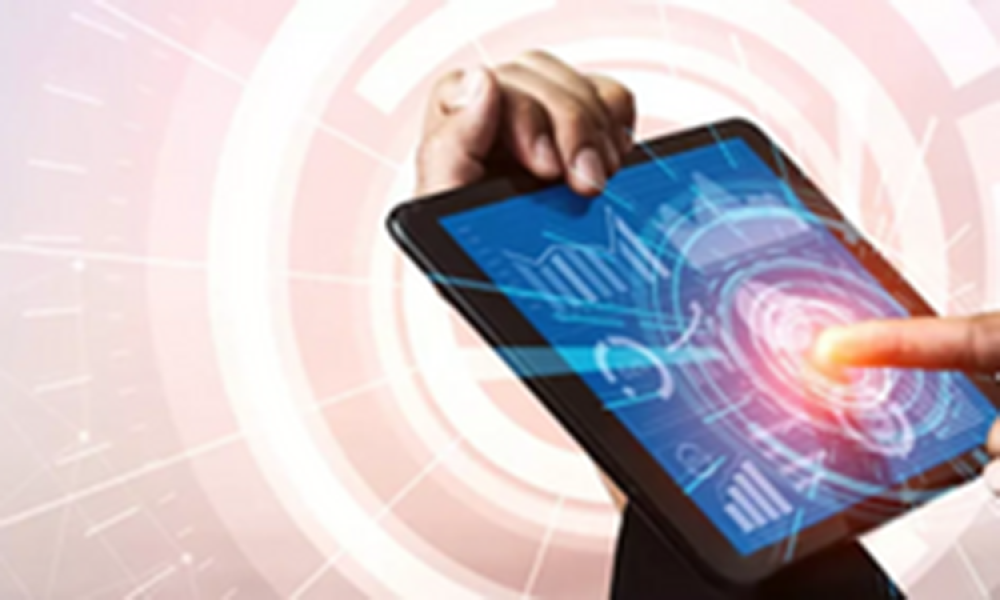
The International Council for Harmonization of Technical Requirements for Pharmaceuticals for Human Use (ICH) has recently announced the adoption of the revised guideline
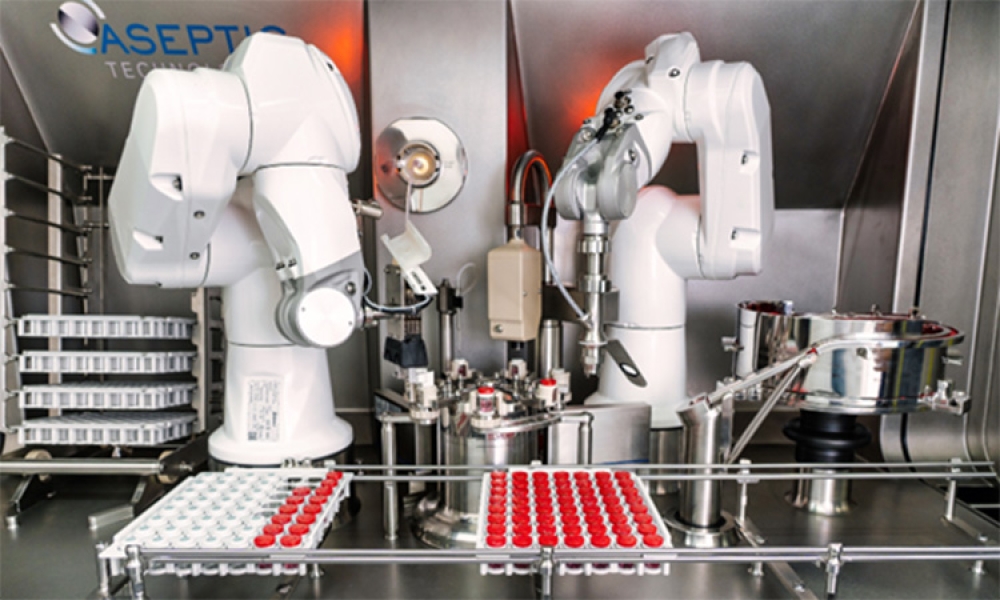
The outlook for the biopharmaceutical market is promising, with expectations that the market will double in the next 10 years, resulting in new therapies and advances in biopharmaceutical manufacturing. This doesn't sound like much at first, but if you consider how the market has developed over the last 20 years, a doubling in the next 10 years is very significant.
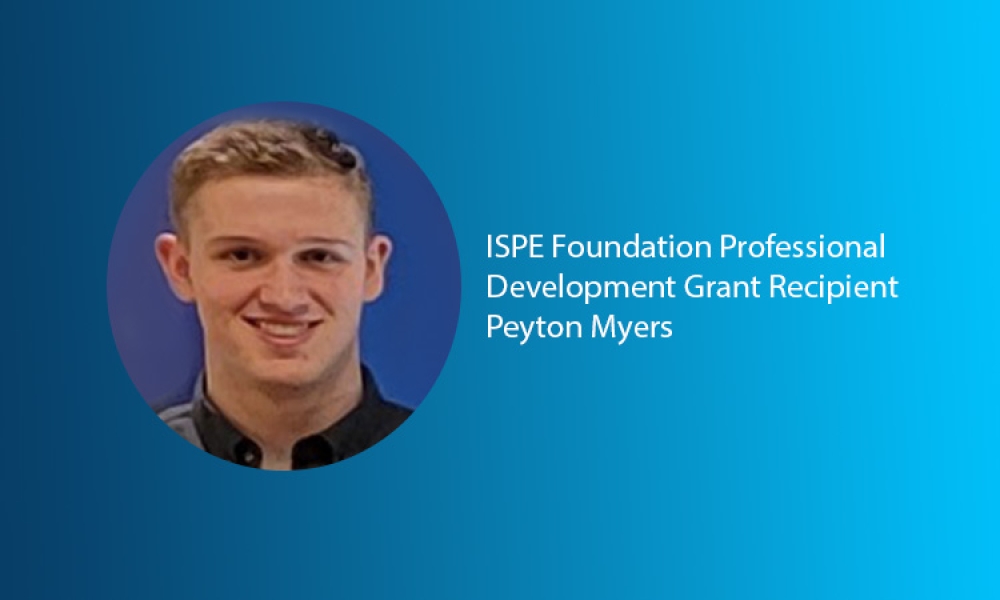
The following blog post was provided by Peyton Myers, an undergraduate student at Appalachian State University. Myers attended the 2023 ISPE Annual Meeting & Expo in Las Vegas as an ISPE Foundation Professional Development Grant recipient.
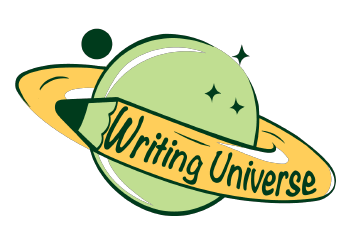
- Environment
- Information Science
- Social Issues
- Argumentative
- Cause and Effect
- Classification
- Compare and Contrast
- Descriptive
- Exemplification
- Informative
- Controversial
- Exploratory
- What Is an Essay
- Length of an Essay
- Generate Ideas
- Types of Essays
- Structuring an Essay
- Outline For Essay
- Essay Introduction
- Thesis Statement
- Body of an Essay
- Writing a Conclusion
- Essay Writing Tips
- Drafting an Essay
- Revision Process
- Fix a Broken Essay
- Format of an Essay
- Essay Examples
- Essay Checklist
- Essay Writing Service
- Pay for Research Paper
- Write My Research Paper
- Write My Essay
- Custom Essay Writing Service
- Admission Essay Writing Service
- Pay for Essay
- Academic Ghostwriting
- Write My Book Report
- Case Study Writing Service
- Dissertation Writing Service
- Coursework Writing Service
- Lab Report Writing Service
- Do My Assignment
- Buy College Papers
- Capstone Project Writing Service
- Buy Research Paper
- Custom Essays for Sale
Can’t find a perfect paper?
- Free Essay Samples
- Corporations
Pharmaceutical Industry
Updated 23 January 2024
Subject Corporations , Marketing , Europe
Downloads 29
Category Business , Economics , World
Topic Company
Summarily, this paper investigates the marketing dynamics that can apply in the European Union pharmaceutical sector in promoting a new medicine. The competition in the pharmaceutical industry in the European Union is intense; hence different companies have to develop unique methods of marketing their products. It is critical to employ the best model to ensure competitiveness in an industry that sees new entrants every time. Notably, it has been identified that pricing strategies are instrumental in ensuring survival of the product in the marketing despite intensive completion from existing medicine. Understanding the best pricing model is critical to the success of a company since many customers will tend to purchase products from companies that have lower rates. Additionally, this research paper discussed the trend in the EU pharmaceutical industry and how it affects companies` intentions to invest in research and development for innovation. The research indicates that companies that are focused on research and development have a better opportunity to have competitive pricing strategies as opposed to other actors in the industry. Other important aspects of this explorative research undertaking are the patent debate and reimbursement and how it impacts pricing strategies adopted by firms as a marketing tool. Companies that can successfully ensure they patent their products develop the best pricing model, which is vital in enhancing increased sales. Moreover, the research recommendations derive important insights on marketing that can assist a manager to apply pricing strategies and innovation as the best weapon to ensure survival in the highly competitive EU pharmaceutical market. The information is critical for new entrants in the industry since they will get a better understanding of the best marketing strategies they can use to attract a substantive customer base.
II. Introduction
The accessibility of health services is a human right issue within the European Union and hence, access to the necessary medicines is a government priority. The pricing system of the pharmaceuticals and the models of supply systems are critical elements in the achievement of the healthcare goals. The current initiatives by WHO on health systems aim at improving access, appropriate use and accessibility of medicine (Bigdeli et al., 2014). European countries in particular, have adopted policies on pricing and funding that are uniform so as to deliver quality medicine to their citizens. The pharmaceutical sector in the European market is regulated intensively as it is viewed to be a health, industrial and budgetary matter that is worthy of attention by all the stakeholders.
The self-medication market has experienced great sales in the EU market causing an alarm, hence necessitating the governments to introduce regulations that are based on the benefits and the possible risks that consumers derive from the medicines. Essentially, increase in the cases of self-medication has led to accessibility of healthcare to a lot of people in the EU but there are legitimate concerns over the misuse of the drugs.
Governmental control on pharmaceutical economics is influenced by factors that are crucial to the wellbeing of the population. Interestingly, the purchase model is not uniform, and the government has to strengthen the moral complexity regarding the right to access healthcare for the European populations. Pharmaceutical marketing has a life cycle in the European market that involves scanning to ensure that it is safe for use and further, an authorization is done for pricing and reimbursement. Additionally, the final stage in the cycle ensures responsible use of the pharmaceuticals to guarantee effectiveness of medicinal products in the long haul.
Nature and Scope of the Problem
The pharmaceutical industry in the EU region has an influential wave that shapes the government policy because of its impact in the achievement of healthcare objectives. Notably, this industry exhibits high levels of competition due to the pressure from various stakeholders on the discovery of new and better medicine. Pharmaceutical sector has over time been identified to have the greatest return on investment around the world.
Pharmaceuticals can be sold over the counter or as prescription drugs which necessitates for the application of an appropriate marketing strategy. The market that should be targeted as a result of the different end users could be medical professionals or the end consumers. The pricing strategy is a common challenge that faces the players in the pharmaceutical industry, especially, during marketing campaigns. The EU and individual governments are critical players in the overall architecture of marketing and authorization of new drugs in this market niche. Normally, EMA has a history of rejecting new medicines which leads to a lot of losses on new entrants in this industry.
This paper will delve on to the methods that are applied by the pharmaceuticals companies in developing pricing systems in a highly competitive environment. The pricing models adopted by the companies have an impact on the brand image, the company`s positioning and the reception by the customers. Information used in this research will be an explorative discussion about academic writings that are relevant to the topic of inquiry. In essence, the best pricing model exploits that balance that is established as a result of forces of demand and supply.
III. Background Information
The pharmaceutical industry in Europe has a great reputation around the world because of the existing track record that emanates from scientific innovation that contributes to discovery of new medicine. This topic was selected for dissertation because of the relevance of the pharmaceutical industry to economic growth of the European countries. A transformative and active drug manufacturing industry has a fundamental influence on trade, healthcare and development of science. Essentially, pharmaceuticals production is promoted by an economy that is knowledge-based.
A manager overseeing a marketing department with plans to introduce new pharmaceutical products in EU region must take into considerations the current dynamics of this industry to adopt the appropriate tools for successful launch. Concerns over investment on a new medicine have been raised by the major players in the industry due to the rising costs on research. Moreover, the regulatory authorities are adopting price austerity measures, while the authorizing agencies are introducing stringent regulations on quality and achievement of health objectives.
Research Objectives
Providing an understanding of the strategic pricing of new pharmaceutical products in the EU.
Providing recommendations to the new entrants about the pricing strategy of products.
Determining how patents influence the pricing of pharmaceuticals in the EU.
IV. Analysis of Trends in the Industry
New drugs are subjected to the rules that regulate the pharmaceutical industry in EU and at the national levels of the respective member states. Under the national competencies there are provisions that relate to allocation of resources, pricing of drugs and medicinal products reimbursement. The issue of ethics in the medicinal drugs corporations has gained a public momentum because many payments are made from the exchequer. The impact of globalization on the pharmaceutical market in EU has led to increased competition, as well as introduction of new opportunities. The manager of new entrants in the EU market should maneuver the rigid regulations to secure the most appropriate price for the product. Noteworthy, medicinal products face unwarranted trade barriers that create uncertainties over the authorization of marketing and pricing of new pharmaceutical products.
Source: Pharmaceutical industry regulators
Essentially, this figure shows in percentages the commitment of various countries to research and development in the pharmaceutical industry.
The pharmaceutical industry has evolved in contemporary times because of intervention by the EU through legislative and non-legislative rules with the ultimate aim of addressing the challenges that make it difficult for industry players to invest in research and innovation. Furthermore, there are major trends in the sector initiated by the governmental agencies to improve the competition policy so as to maintain the integrity of the pharmaceuticals market. ”Transparency Directive” frameworks have been developed with the objective of making the national pricing models better and open to public scrutiny (Babar, 2015). Understandably, the regulations by the various agencies are a trend that is necessary so as to ensure that drugs are viable economically and by adding of therapeutic gains to the users.
Analysis of Industry Trends from a Governmental Perspective
The issue of drug prices and reimbursement is a dicey matter in EU member states because it influences accessibility of medicine to patients due to factors such as affordability and availability. The appraisals for new drugs that are in line for launching have to comply with the different national regulations of the EU member states. The adoption of an external price referencing is not desirable as an industry trend because pharmaceutical companies introduce their product at a lower price in a particular market segment with the hope that they will increase it in another market.
Figure 1 above illustrates the major regions that engage in the pharmaceutical industry in the region. It is evident that Germany has a wider share as manufacturers; hence, they are at an upper hand in creating pricing strategies in the industry.
Policy changes that have been introduced via Lisbon Treaty gave EU a mandate to monitor the health systems of the member countries. Noteworthy, the EU policy instrument that have been adopted as a trend for the pharmaceutical market have made it attractive for patients to buy alternative generics so as to save costs. Healthcare in EU countries is usually a governmental responsibility that is implemented through a partnership with the insurance companies and any action taken at the regional level is meant to complement the national policies of respective countries. However, the issue of brexit has had a significant impact in the manner in which the EU countries relate in the pharmaceutical industry. It is difficult to develop policies that suit all countries in the European Union. The United Kingdom, for instance, is not part of the policies made by the EU (Lorgelly, 2018). Brexit has affected the pharmaceutical industry in a negative way since it is difficult to develop policies that are embraced by all the member countries (Song, 2016). It has affected the development of binding laws and regulations.
Essentially, in contemporary times manufacturers that have launched new pharmaceutical products enjoy some level of exclusivity on their patents thus enabling them to set prices that will cover all the costs incurred. The costs that are incurred in producing new pharmaceutical products have increased in modern times and the manufacturing companies are unable to assess them accurately (Enzmann, H., 2016). A scope of the regulations have been initiated by the governmental agencies in order to address the factors that lean on the demand side, consisting of patients and medical professionals, and the supply side that addresses market elasticity and the pharmaceuticals. The EU health laws and policies have been critical in establishing an industry that is guided by common regulations (Hervey et al., 2017). It becomes difficult for different companies to hike prices of pharmaceuticals since there is a set standard; hence, reducing monopoly and unfair competition.
V. Methodological Approach
The method applied in this research paper is exploratory because it involves an analysis of academic enquiries that have been conducted in this field. The data sources that are used in the paper are mainly reports from governmental agencies and reputable scholars that have an interest in the topic being discussed. In essence the justification of the methodology stems from the need to understand from a policy perspective the hurdles and opportunities that present for drug discoverers as they seek to gain market entry into the EU.
Essentially, an integral part of the research methodology that was applied in this study was a skimming of the pharmaceutical policies that have been introduced within the EU framework to control pricing and purchasing of medicines. The academic sources used in this review are seminar papers that have relevant information on the topic and other authoritative policy documents that have been influential in the development of legislations and regulations in the pharmaceutical industry. The scope of this explorative research is broad covering all aspects that have an impact on the development of a pricing strategy for a medicinal product in the EU market. Additionally, there is inclusion of the multi-dimensional nature of pharmaceutical marketing norms so as to come up with recommendations that can assist new entrants in setting the most appropriate prices (Hanf, 2011). The key phrases that are critical in this research paper include reimbursement policies, price regulations and market authorization.
Notably, the explorative study investigates the impact of generic medicine in influencing drug pricing policies at the country level. The issue of competition between the generics and the branded drugs that have IP rights has a bearing on the pricing strategies as different member states of the EU and their different regulations. The regulatory regimes of EU countries have been considered extensively in developing recommendations for a company that is a new entrant in this market niche.
Generic drugs are usually copies of drugs that have already been branded and they accomplish the same treatment goal that is intended by the manufacturers of the branded medicine. The competition challenge that is existent in the European market stems from the issue of generic and brand drugs in which the latter has gained prominence among the consumers due to their affordability. The non-patented pharmaceuticals have been problematic because they interfere with the market prices. The market for generic medicine has expanded significantly in the EU market contributing about half of the consumed pharmaceutical products. Governments are facing challenges on promoting the use of generic medicine to promote access to healthcare while at the same time ensuring that are innovations in the pharmaceutical industry (Herr, and Suppliet, 2012).
This paper studies diverse methods for pricing of new pharmaceuticals on the European market. This includes medicines developed for humans, without pharmaceutical equipment. Different strategies will be discussed along with their consequences for the selling company regarding finances, customers and brand image. Before jumping into further detail, there are a couple of concepts that need to be explained.
Porter`s 5 Forces of Analysis
The pharmaceutical industry has a lot of stakeholders that have vested interest and in some other cases power to control the market dynamics and the prices. Pharmaceutical companies have to invest on stakeholder management so as to address various interests that interplay in negotiating for prices and ensuring that customer needs are satisfied. The pharmaceutical industry is based on innovation and the pricing system is hinged on the competition policy. European Union enforces the authorization regulations for drug manufacturing companies within the body`s jurisdiction. Pharmaceutical companies in contemporary times have to satisfy the perceptions on value from stakeholders such as payers to be given access to markets (Sendyona et al., 2016). The growth and factors that influence economic success of companies operating in the pharmaceutical sector depend on the level of innovation and the investments on research and development. The marketing campaigns of pharmaceutical manufacturers should have clear and concise messages that appeal to the needs of the customers.
A new entrant in the pharmaceutical industry in Europe should consider factors relating to their customer base, the competitors and the capacity of the organization to position itself in a particular market niche. Noteworthy, a new pharmaceutical organization must establish clarity in terms of ensuring that it possesses a differential advantage and a clear understanding of the target market. The products offered by a drug manufacturing organization must be memorable to the consumers in order to create a relationship that develops to loyalty (Vogler and Martikainen, 2015. The differential advantage that is enhanced by the introduction of new pharmaceutical product has to be credible from the analysis of a consumer. Additionally, the innovation introduced in new products is supposed to give the companies a competitive edge in the market.
Competition in the Industry
The pharmaceutical sector in Europe exhibits intensive competition and the participants are in the private sector, as well as government agencies. The factors that influence competition are price, reliability of the products and customer service. Claim to patents and the manufacturing rights guarantee the new entrants a chance for survival in the market. Changes in the industry because of acquisitions and mergers have an influential role in the development of the pharmaceutical sector (Margaret, 2007). A new entrant in a highly rigid market in terms of regulation should have distinctive advantages over other competitors.
The critical element that is valuable for new entrants in the pharmaceutical industry lies in the development of a branding policy that creates an identity for a product. Customers are attracted to drugs that have closer associations and a strong perception on quality (Fernandez Utges Manley, 2015). The position of a new product is important because it is hinged on consumer needs and preferences, an undertaking that can assist a corporation to dominate the market.
The power of the suppliers
Pharmaceutical companies have to put into considerations various aspects of the market they ventures are located before implementing pricing strategies. Essentially, to address the pricing challenges new entrants can create market segments with the aim of offering products that address the specific needs of a particular group in a population.
Substitutes
The main concern for the pharmaceutical market is the existence of substitutes because they have the capacity to devalue the branded products hence creating a risk of vulnerability. Furthermore, the impact of globalization in the pharmaceutical industry has led to an increase in the manufacturers in the hope of reaping super profits. The aspect relating to expiry of patents is problematic because it encourages the production of generics that compete with the branded drugs. Pharmaceutical corporations have a responsibility to capitalize on the opportunities that are induced by the influence of globalization and neuter threats that are posed by the saturation of the markets (Colak, 2014). Launching new branded drugs demands an introduction of a marketing infrastructure that will aid in the building of a reputation for the brand. The competition from the herbal drugs can be quelled mainly due to their inability to prevent health conditions such as cancer.
PESTEL Analysis
1) Political
Politicians on their part view of process of pricing for the pharmaceuticals to be exploitative and a preoccupation of cartels whose intention is to profit from the public. The companies are forced to engage a wide array of stakeholders to understand the complex process of pricing that is primarily determined by valuation. Pricing attracts political attention; hence, becoming a source of debate on the issue (Löfgren, 2017). The pharmaceutical industry is attracting attention from the policy makers because of its role in the achievement of the healthcare goals. Notably, healthcare services have become expensive and this is a responsibility that EU countries national governments have taken as a burden that over time has morphed to be an election agenda. The repercussions on the industry are stringent measures by governments that are under pressure to deliver on healthcare by the citizens.
2) Economical
The high competition levels in the pharmaceutical industry has contributed to innovation leading to introduction of new products and market entrants that are facing hurdles in gaining foothold in the sector. However, more companies have been established in the industry and there is a growing portfolio of the products in this segment and eventually the economic benefits have been extended to the public through buying shares and securities from the listed drug manufacturers.
The social aspect of pharmaceutical companies in the EU region is mainly on the commitment to their corporate responsibility. The mainstay of pharmaceutical companies is the achievement of a social need by delivering quality healthcare. Drug manufacturing companies are more responsive to the issues in the society by driving innovations that contribute to wellbeing of the society. Drugs manufacturing companies must adhere to established systems of social accountability, corporate compliance, philanthropy and ethics.
4) Technological
Innovation has been a major driver of developments in drug manufacturing sector in the EU region due to the pressure to develop better drugs and the competition emerging from the generics leading to reduced prices of branded medicine. Undoubtedly, the EU market is highly competitive and industry players have to invest heavily on research and innovation so as to remain relevant and come up with products that address the contemporary health challenges. Companies have a risk of being defined as venerable in case the health products they produce are inferior compared to those offered by the competitors.
5) Environmental
The aspect of compliance in the pharmaceutical sector has been reinforced because of the underlying factors relating to customer preference by demanding quality and a fair pricing for medicines. Consumers of the products solely depend on the trust that is established between them and the regulatory authority to protect their interest in the sector. The overhead costs that are associated with the regulation and compliance requirements reduce the investments that are directed towards innovation. The research initiatives undertaken by the companies in the pharmaceutical industry are focusing on the aging generation because they are biggest consumers of the drugs.
The political environment and the society in general have great influence in the functionality and operations conducted by pharmaceutical companies, especially on matters ethics. However, regulatory authorities have greater mandate in ensuring that there is compliance with the laws for consumer protection. Essentially it is incumbent upon the company to demonstrate its compliance to safety standards and the effectiveness of administering the drugs manufactured on the patients.
VI. Contemporary Pricing Strategies in the Pharmaceutical Industry
Drug pricing has remained as a contentious issue that has affected the entry of new players in the EU market due to the risk of intensive competition from well-established companies. Noteworthy, price strategies in this unique market are affected by the market forces, intensive political scrutiny and pricing regulations that discourage introduction of new products because of the rigidity of market entry (Aaker and McLoughlin, 2010). In essence, developing the appropriate pricing system is critical because it will offer immense value. Drug manufacturers are facing a lot of challenges in contemporary times, especially with regard to the introduction of new drugs to improve the standard of care with no peer to support the evaluation. It is; therefore, critical for companies to develop effective marketing approach in the industry (Lidstone and MacLennan, 2017). Having a sound marketing plan is vital sine it will increase the customer base of the pharmaceutical products.
Determination of the pricing for a pharmaceutical product
The contemporary times pricing norms for new products in the pharmaceutical industry are determined by their effectiveness in terms of therapeutic gains to the consumer. The main determinant that eventually leads to price wars is the therapeutic advance that embodies a drug that is introduced in the market (Carone et al., 2012). Drugs that have greater therapeutic results on the patients may have their prices twice those of comparable levels. Competitive pressures play a significant role in terms as launch prices are influenced by the pricing of the substitutes. Duplicate products such as generic drugs are essential in the development of pricing strategies for the pharmaceutical industry.
Pharmaceutical company have caused a media uproar due to increase in the prices of drug which is not related to the rate of inflation. In essence, there are numerous issues that affect the pricing system that is adopted by EU countries in an effort to control the pharmaceutical industry. The services of an auditor are in most cases necessary in determining the unfair practices that could be sanctioned by stakeholders in the industry. The pricing strategy in the EU has had to be developed effectively to ensure there is fairness in the market (Faulkner et al., 2016). It has also been a key strategy used by companies to ensure they have a competitive edge in the market.
Pricing strategies have a major impact on timing that launch of new products is done and the reception by the target market. Countries that institute price controls in their market make it difficult for external players and new entrants to gain entry into the EU pharmaceutical industry (Margaret, 2007). The legal framework has also made the pricing regime in the EU regime complicated because of allowing the import of parallel substitutes. Price regulation practices in one region affects the pricing strategies of the domestic firms and entry of external investors in the market.
Pricing Regulations
Price change is impactful on supply of drugs within the EU and the method applied in reimbursement influences the relationship and the memorability of a particular brand. Essentially, a cross-reference pricing system is adopted because it is agreeable among the decision makers. Furthermore, there are greater incentives with regard to strong incentives that are introduced due to effect of spill-overs as a result of impact by foreign drug prices (Vogler and Martikainen, 2015). However, to alleviate the problems that are associated with cross-referencing in pricing and index that considers all the countries should be created in determination of the definite price for reimbursement.
Challenges facing pharmaceutical industries in percentages
Source: EU policy documents.
Figure 2 provides an overview of the challenges players in the pharmaceutical industry face. The major problem is increased manufacturing cost, which influences the pricing of the products. Additionally, the increased customer expectation to lower the prices of the products also forms part of the big challenges the industry faces. It becomes difficult to balance between the customer expectation and the profitability of the company.
Introducing a new pharmaceutical product in a market like EU that adopts a differential pricing system can be transformative because it gives incentive for the innovators that introduce new drugs in the market. The dicey issue that investors in the pharmaceutical industry is the inability to recover costs that relate to research and development (Ruggeri and Nolte, 2013). In Europe, the prices of the pharmaceuticals are decided at the national government`s level. Drugs are provided as a form of subsidy which is supposed to be reimbursed by the payers. In case, the health insurance is introduced by a company that enjoys monopoly, then, there is high likelihood that it will influence the drug prices. In essence, the manufacturers do not have the opportunity to negotiate the prices of the medicines in the various countries within the EU framework (Simoens, 2012). Notably, adopting profit maximization strategies can only be effective with delayed distribution of innovative medicines or ultimately avoiding their access which affects access of healthcare impacting on welfare. The patients in a very competitive market for pharmaceuticals are denied access to new medicine.
The regulatory pathway for the European Union is developed by the European Union Medicines Agency (EMEA) that has legislative authority with regard to allowing industry players to introduce new products. New market entrants have to be aware of the existing pricing systems and they have to negotiate with the national governments and agree on a refund rate. Essentially, there is a determination of the additional benefits that patients will derive from the new drug to another comparative product that is expedient (Kyle, 2007). The negotiations for refund rates are negotiated in case there is an identifiable benefit that will accrue to consumers by using a particular pharmaceutical product compared to another. Noteworthy, the pricing strategy for a new entrant is determined by their ability to come up a with a product differentiation that will lead to therapeutic benefits for the consumers. A pharmaceutical company that introduces a medicine with additional benefits has a legitimate basis to charge a higher price as part of the comparative therapy.
VII. Challenges Facing New Pharmaceuticals in EU Market
New pharmaceutical companies in the European market face great challenges due to the rigidity of the regulations and the complexities of the pricing systems. In essence, an additional benefit emanating from investment in innovation will guarantee a new entrant in the pharmaceutical industry a greater power in the negotiation of a higher price compared to other substitutes in the market. The major issue of contention that is existent between entrant pharmaceutical companies and the regulatory authorities stems from the selection of a comparative therapy that is an under-priced generic product (Cravens and Glover, 1995). Market authorization and pricing is approved upon evaluation of the dossiers that are delivered by the pharmaceutical companies with the ultimate aim of ensuring that the consumer is protected from exploitation by the manufacturers.
The price negotiation for drug discoverers as players in the pharmaceutical sector should start in initial stages so as to define the impact expected from a project and the end points that will be realized. The trial design is critical in the determination of the pricing and the model reimbur
Deadline is approaching?
Wait no more. Let us write you an essay from scratch
Related Essays
Related topics.
Find Out the Cost of Your Paper
Type your email
By clicking “Submit”, you agree to our Terms of Use and Privacy policy. Sometimes you will receive account related emails.

An official website of the United States government
The .gov means it’s official. Federal government websites often end in .gov or .mil. Before sharing sensitive information, make sure you’re on a federal government site.
The site is secure. The https:// ensures that you are connecting to the official website and that any information you provide is encrypted and transmitted securely.
- Publications
- Account settings
Preview improvements coming to the PMC website in October 2024. Learn More or Try it out now .
- Advanced Search
- Journal List
- Innov Pharm
- v.13(2); 2022

Artificial Intelligence (AI) in Pharmacy: An Overview of Innovations
Muhammad ahmer raza.
1 Department of Pharmacy Practice, The University of Lahore, Punjab, Pakistan
2 Faculty of Pharmacy, The University of Faisalabad, Punjab, Pakistan
Shireen Aziz
3 School of Pharmacy, Zhengzhou University, Henan, China
4 Faculty of Pharmacy, University of Sargodha, Punjab, Pakistan
Misbah Noreen
5 School of Pharmacy, University of Agriculture, Faisalabad, Punjab, Pakistan
6 Department of Pharmacy Administration and Clinical Pharmacy, School of Pharmacy, Xi’an Jiaotong University, Xi’an, China
7 Center for Drug Safety and Policy Research, Xi’an Jiaotong University, Xi’an, China
Irfan Anjum
8 Faculty of Pharmacy, The University of Lahore, Pakistan
9 Faculty of Pharmacy, Hacettepe University, Ankara, Turkey
Mudassar Ahmed
Shahid masood raza.
10 School of Pharmacy, Tongji Medical College, Huazhong University of Science and Technology, Hubei, China
Artificial Intelligence (AI) emerged as an intervention for data and number-related problems. This breakthrough has led to several technological advancements in virtually all fields from engineering to architecture, education, accounting, business, health, and so on. AI has come a long way in healthcare, having played significant roles in data and information storage and management – such as patient medical histories, medicine stocks, sale records, and so on; automated machines; software and computer applications like diagnostic tools such as MRI radiation technology, CT diagnosis and many more have all been created to aid and simplify healthcare measures. Inarguably, AI has revolutionized healthcare to be more effective and efficient and the pharmacy sector is not left out. During the past few years, a considerable amount of increasing interest in the uses of AI technology has been identified for analyzing as well as interpreting some important fields of pharmacy like drug discovery, dosage form designing, polypharmacology, and hospital pharmacy. Given the growing importance of AI, we wanted to create a comprehensive report which helps every practicing pharmacist understand the biggest breakthroughs which are assisted by the deployment of this field.
Introduction
AI is a stream of science related to intelligent machine learning, mainly intelligent computer programs, which provides results in a similar way to the human attention process [ 1 ] . This process generally comprises obtaining data, developing efficient systems for the uses of obtained data, illustrating definite or approximate conclusions, self-corrections, and adjustments [ 2 ] . In general, AI is used for analyzing machine learning to imitate the cognitive tasks of individuals [ 2 , 3 ] . AI technology is exercised to perform more accurate analyses as well as to attain useful interpretation [ 3 ] . In this perspective, various useful statistical models, as well as computational intelligence, are combined in AI technology.
Recently, AI technology becomes a very fundamental part of the industry for useful applications in many technical and research fields. Reflecting on the past 25 years, pharmacy has done a great job of addressing the growing demand for prescriptions, even when faced with pharmacist shortages, growing operating costs, and lower reimbursements. Pharmacy has also done a great job of leveraging enabling technology automation to improve workflow efficiency and lower operating costs while promoting safety, accuracy, and efficiency in every pharmacy setting. Automated dispensing gives pharmacists more time to engage with a greater volume of patients while also enhancing their health outcomes [ 4 ] .
The first application of a computer in a pharmacy presumably dates back to the 1980s and since then, computers have been utilized in everything from data collection, retail pharmacy management, clinical research, drug storage, pharmacy education, clinical pharmacy, and lots more, and with the emergence of artificial intelligence, there is no telling just how much the Pharmacy sector will evolve in the long run. There have been several expert systems developed in medicine to assist physicians with medical diagnosis [ 5 ] . Recently, several programs focusing on drug therapy have been described [ 6 ] . They guide drug interactions, drug therapy monitoring, and drug formulary selection. There are many aspects of pharmacy that AI can have an impact on and the pharmacists to consider these possibilities because they may someday become a reality in pharmacy practice.
The purpose of this article was to review topics related to AI. The topics include AI general overview and classification, AI uses in hospitals, the pharmaceutical industry, and retail pharmacies and to create awareness for AI as a component of pharmacy practice in the future, to encourage pharmacists to embrace this advancement, and as much as possible put in the effort to acquire the relevant skills, which will enable pharmacists to contribute towards the much-envisaged development.
AI general overview
The term AI (also known as machine intelligence) is very commonly confused and used interchangeably with robotics and automation. While robotics is simply the creation of machines that can carry out difficult repetitive tasks, AI refers to the exhibition of human-like behaviors or intelligence by any computer or machine [ 7 ] . Traditionally, robots were not built to possess these “intelligent capabilities” even though they may be able to move or carry objects independently using a designed program and surface sensors in a process known as automation. AI, in essence, is the field of computer science that specializes in the creation of intelligent machines, developed with the ability to perform tasks that will ordinarily be associated with a human being [ 8 ] .
AI is frequently applied to the development of digital computers or computer-controlled robots with the capacity to autonomously execute intellectual and cognitive human-like processes. Such intellectual and cognitive processes include learning, reasoning, problem-solving, perception, and language. The form of AI currently in use today is referred to as narrow AI or weak AI because it is only designed to perform narrow tasks like internet search, facial and voice recognition, controlling and driving cars, and so on. However, the long-term goal of the AI community is to have machines that can autonomously outperform humans’ at all cognitive tasks. The AI that involves creating machines that can perform all human cognitive tasks will be the general AI or Strong AI (ADI) [ 9 ] .
In simple terms, AI refers to the ability of machines and computers to think, act, behave and function as human beings. Familiar examples of AI-controlled systems include Apple’s SIRI (in iPhone) [ 10 ] , Amazon’s Alexa [ 11 ] , and the self-driving cars of Google, Mercedes, BMW, and Tesla to name a few [ 12 ] . The core of AI can be Knowledge Engineering, in which machines are assembled with access to abundant data and information relating to the human world, which enables them to be able to mimic human behavior. Machine Learning is another type of AI, which involves the use of algorithms and statistical models to improve the accuracy of software applications in predicting outcomes without being distinctly programmed. It was established based on the idea that machines can learn from data, identify problems and make decisions with minimum human help or intervention. Applications of machine learning include self-driven Google cars, fraud detection, and online recommendation offers like those on Amazon and Netflix [ 13 ] . Machine perception is another aspect of AI and it involves designing and building machines with the ability to use sensory inputs to deduce information about the different aspects of the world. Computer vision is the ability of machines to process visual inputs such as facial information, objects, and gestures [ 14 ] .
There have been various skepticism, criticism, and myths towards AI mostly concerning safety and the dangers that may be potentiated by the creation of machines that could match human cognitive capabilities. One of the five predictions made by Forbes for AI in 2019 [ 15 ] is that it may become an issue of national politics. Aside from concerns that AIs may be used as weapons for war and mass destruction, certain people have expressed concerns that the creation of AI systems that are smarter than humans, through general AI could be more fatal and be the end of the human race itself. They believe we may not be able to predict how AI systems that are more intelligent than us will behave and that humans may end up being controlled by these super-intelligent machines. Scientists believe most of the safety concerns about future super-intelligent AI systems may be resolved if the “goals” of these machines can be made to align with our own goals [ 15 ] .
AI classification
AI can be classified in two different ways [ 16 , 17 ]
- a) according to caliber
Classification of AI
Based on their caliber, AI system is classified as follows:
- Weak intelligence or Artificial narrow intelligence (ANI) : This system is designed and trained to perform a narrow task, such as facial recognition, driving a car, playing chess, and traffic signaling. E.g.: Apple SIRI virtual personal assistance, tagging in social media.
- Artificial General Intelligence (AGI) or Strong AI : It is also called Human-Level AI. It can simplify human intellectual abilities. Due to this, when it is exposed to an unfamiliar task, it can find the solution. AGI can perform all the things as humans.
- Artificial Super Intelligence (ASI): It is brainpower, which is more active than smart humans in drawing, mathematics, space, etc; in every field from science to art. It ranges from the computer just little than the human to a trillion times smarter than humans.
Arend Hintze [ 18 ] , an AI scientist classified the AI technology based on its presence and not yet present. They are as follows:
- Type 1: This type of AI system is called a Reactive machine. E.g. Deep Blue, the IBM chess program which hit the chess champion, Garry Kasparov, in the 1990s. It can identify checkers on the chessboard and can make predictions; it does not have the memory to use past experiences. It was designed for narrow purposes use and is not useful in other situations. Another example is Google’s AlphaGo.
- Type 2: This type of AI system is called a Limited memory system. This system can use past experiences for present and future problems. In autonomous vehicles, some of the decision-making functions are designed by this method only. The recorded observations are used to record the actions happening in the future, such as changing the lanes by car. The observations are not in the memory permanently.
- Type 3: This type of AI system is called as “theory of mind”. It means that all humans have their thinking, intentions, and desires which impact the decisions they make. This is a non-exist AI.
- Type 4: These are called self-awareness. The AI systems have a sense of self and consciousness. If the machine has self-awareness, it understands the condition and uses the ideas present in others’ brains. This is a non-existing AI.
Applications of AI
Ai in diagnosis and targeted genomic treatments.
There are several applications of AI in hospital-based health care systems [ 19 , 20 ] in organizing dosage forms for individualized patients and selecting suitable or available administration routes or treatment policies.
- Maintaining of medical records: Maintenance of the medical records of patients is a complicated task. The collection, storage normalizing, and tracing of data are made easy by implementing the AI system. Google Deep Mind health project [ 21 ] (developed by Google) assists to excavate the medical records in a short period. Hence, this project is a useful one for better and faster health care. The Moor fields Eye hospital NHS is assisted by this project for the improvement of eye treatment.
- Treatment plan designing: The designing of effective treatment plans is possible with the help of AI technology. When any critical condition of a patient arises and the selection of a suitable treatment plan becomes difficult, then the AI system is necessary to control the situation. All the previous data and reports, clinical expertise, etc., are considered in the designing of the treatment plan as suggested by this technology. IBM Watson for Oncology [ 22 ] , the software as a service, is a cognitive computing decision support system that analyzes patient data against thousands of historical cases and insights gleaned from working thousands of hours with Memorial Sloan Kettering Cancer Center physicians and provides treatment options to help oncology clinicians make informed decisions. These treatment options are supported by literature curated by Memorial Sloan Kettering, and over 300 medical journals and 200 textbooks, resulting in almost 15 million pages of text [ 22 ] .
- Assisting in repetitive tasks: AI technology also assists in some repetitive tasks, such as examining the X-ray imaging, radiology, ECHO, ECG, etc., for the detection and identification of diseases or disorders. Medical Sieve [ 23 ] (an algorithm launched by IBM) is a “cognitive assistant” having good analytical and reasoning abilities. A medical start-up is necessary for the improvement of the patient’s condition by combining deep learning with medical data. A specialized computer program is available for each body part and used in specific disease conditions. Deep learning can be employed for almost all types of imaging analyses, such as X-ray, CT scan, ECHO, ECG, etc.
- Health support and medication assistance: In recent years, the uses of AI technology are recognized as efficient in health support services and also, for medication assistance. Molly [ 24 ] (a start-up-designed virtual nurse) receives a pleasant voice along with a cordial face. Its aim of it is for helping patients to guide the treatment of patients as well as support them with their chronic conditions during doctor’s visits. Ai Cure [ 25 ] is an app existing in a Smartphone webcam, which monitors patients and assists them to control their conditions. This app is useful to patients with severe medication situations and for patients who participate in clinical trials.
- Accuracy of medicine: AI shows a good impact on genomics and genetic development. Deep Genomics [ 26 ] , an AI system is useful for observing patterns in the genetic information and medical records to identify the mutations and linkages to diseases. This system informs doctors about the events happening within a cell when DNA is altered by genetic variation. An algorithm is designed by the father of the human genome project, Craig Venter [ 27 ] that gives information on patients’ physical characteristics based on their DNA. “Human Longevity” AI technology is useful to identify the exact location of cancer and vascular diseases in their early stage.
- Drug creation: The development or creation of pharmaceuticals takes more than a decade and consumes billions of rupees. “Atomwise” [ 28 ] , an AI technology that uses supercomputers, is useful to find out the therapies from the database of molecular structure. It hurled a virtual search program for safe and effective therapy for the Ebola virus with the existing drugs. The technology identified two drugs that caused Ebola infection. This analysis was completed within one day compared to months to years with manual analysis. A Biopharma company in Boston developed big data for the management of patients. It reserves data to find the reasons why some patients survive diseases. They used patients’ biological data and AI technology to find out the difference between healthy and disease-friendly atmospheric conditions. It helps in the discovery and design of drugs, healthcare, and problem-solving applications.
- AI helps people in the health care system: The “open AI ecosystem” [ 29 ] was one of the top 10 promising technologies in 2016. It is useful to collect and compare the data from social awareness algorithms. In the healthcare system, vast information is recorded which includes patient medical history and treatment data from childhood to that age. This enormous data can be analyzed by the ecosystems and gives suggestions about the lifestyle and habits of the patient.
- Healthcare system analysis : In the healthcare system, if all the data is computerized then retrieval of data is easy. Netherland maintains 97% of invoices in digital format [ 30 ] , which contain treatment data, physician names, and hospital names. Hence, these can be retrieved easily. Zorgprisma Publiek, a local company analyses the invoices with the help of IBM Watson cloud technology. If any mishap occurs, it recognizes it immediately and takes the correct action. Because of this, it improves and avoids patient hospitalization.
AI and development of pharmaceuticals [ 31 - 37 ]
Top pharmaceutical companies are collaborating with AI vendors and leveraging AI technology in their manufacturing processes for research and development and overall drug discovery. Reports show nearly 62 percent of healthcare organizations are thinking of investing in AI shortly, and 72 percent of companies believe AI will be crucial to how they do business in the future. To get a better sense of the future of AI in the sector, Pharma News Intelligence [ 38 ] dives into current AI use cases, the best uses for the technology, and the future of AI and machine learning. The McKinsey Global Institute estimates that AI and machine learning in the pharmaceutical industry could generate nearly $100B annually across the US healthcare system. According to researchers, the use of these technologies improves decision-making, optimizes innovation, improves the efficiency of research/clinical trials, and creates beneficial new tools for physicians, consumers, insurers, and regulators. Top pharmaceutical companies, including Roche, Pfizer, Merck, AstraZeneca, GSK, Sanofi, AbbVie, Bristol-Myers Squibb, and Johnson & Johnson have already collaborated with or acquired AI technologies. In 2018, the Massachusetts Institute of Technology (MIT) partnered with Novartis and Pfizer to transform the process of drug design and manufacturing with its Machine Learning for Pharmaceutical Discovery and Synthesis Consortium [ 38 ] .
Research works are carried out daily to find new active principles for the currently incurable diseases and conditions; increase the safety profile of already existing drugs; combat drug resistance and minimize therapeutic failure. Hence, there is an increase in the size and variety of biomedical data sets involved in drug design and discovery. This factor and many more contributed to the advancement of AI in the pharmaceutical industry. Today, some companies offer software with much relevance in drug design and data processing, as well as in predicting treatment outcomes.
GNS healthcare [ 39 ] uses AI machine software known as Reverse Engineering and Forward Simulation (REFS). REFS determines the cause and effect relationships between various types of data, that are unforeseen ordinarily by direct data evaluation. GNS claims that REFS can transfer millions of data points ranging from clinical to genetics, laboratory, imaging, drug, consumer, geographic, pharmacy, mobile, proteomic, and so on. In drug design, a company known as Atomwise developed the first deep learning neural network for structure-based drug design and discovery that they called AtomNet [ 40 ] . AtomNet makes use of a statistical approach to extract information from millions of experimental affinity measurements and thousands of protein structures to predict the binding properties of small molecules with proteins. By presenting 3-dimensional images of the protein and ligand pair showing channels for carbon, oxygen, nitrogen, and other types of atoms, AtomNet technology enables the pharmaceutical chemists to perform core processes of drug discovery and design like hit discovery, lead optimization, and prediction of toxicity with high precision and accuracy in weeks as against years.
Insilico Medicine [ 41 , 42 ] announced an AI project by the company called Pharm AI. Insilico Medicine claims they applied Generative Adversarial Networks (GAN) and reinforcement learning algorithms. The GAN is a type of generative model that can generate samples and also learn from training samples. They are made up of two neural networks, the generator, and the discriminator. The relationship between the generator and the discriminator is referred to as “adversarial”. The generator tries to create and learns to create new samples and sends them to the discriminator, which classifies the sample as real or fake where real denotes the examples that belong to the data set, and the examples generated by the generator are denoted “fake”. Through continuous training, the generator begins to create samples that are similar to the real ones while the discriminator gets better at the identification process. With Pharm AI, through GAN and reinforcement learning, Insilico Medicine claims that it can generate new molecular structures and ideate the biological origin of a disease.
AI in pharmacy practice in hospital and community pharmacies
Machine learning models allow e-mails to be personalized at a speed and accuracy greater than that of any human being. Chatbots [ 43 ] can be used to increase the efficiency of service delivery. Chatbots are capable of mimicking interactions between customers and customer care of sale staffs. Chatbots are capable of automatically resolving customer complaints and queries and the difficult questions are transferred to human staff. In retail pharmacy, this principle can be applied. The chatbots can be programmed to mimic pharmacist-patient interaction.
Walgreen [ 44 ] made a partnership with Medline, a telehealth firm to create an avenue to help patients interact with healthcare professionals through video chat. AI can also be useful in inventory management. As a retail pharmacist, imagine being able to predict what your patients will need in the nearest future, stocking them, and using personalized software to deliver e-mails to remind the patient of drug needs. With the use of AI-powered data analytics, a patient’s future drug purchase can be predicted. Predicting the patient’s drug purchase through AI will help the pharmacist to make proper stock procurement decisions.
Although, there are existing inventory management software and application that are used in retail pharmacy stock management like Mckessons; Liberty; Winpharm; PrimeRx; and WinRx, not all of them utilize AI or machine learning. For example, an AI company, Blue Yonder developed software for Otto group [ 45 ] , a German online and catalog retailer. This software can predict with 90% accuracy what will be sold by Otto in 30 days. This reduced the delivery schedule for purchased products from one week or more to one of two days by enabling direct delivery of the product from the supplier to the consumer without having to pass through the warehouse.
Intending to improve the safety of patients, the University of California San Francisco (UCSF) Medical Center uses robotic technology for the preparation and tracking of medications. According to them, the technology has prepared 3, 50, 000 medication doses without any error. The robot has proved to be far better than humans both in size as well as its ability to deliver accurate medications. The abilities of the robotic technology include the preparation of oral as well as injectable medicines which include toxic chemotherapy drugs. This has given the freedom to the pharmacists and nurses of UCSF so that they can utilize their expertise by focusing on direct patient care and working with the physicians. Within the automated system of the pharmacy, the computers first receive medication orders electronically from the physicians and pharmacists of UCSF. After this, individual doses of pills are picked, packaged, and dispensed by the robotics. This is followed by machines assembling the doses onto a bar-coded plastic ring. The thin plastic ring contains all medications that have to take by a patient within a period of 12h. Adding to the capabilities of the automated system is their ability to prepare sterile preparations that are meant for chemotherapy along with filling intravascular syringes with the right medications [ 46 ] .
The primary aim of health-related AI applications is to analyze relationships between prevention or treatment techniques and patient outcomes. AI programs have been developed and applied to practices such as diagnosis processes, treatment protocol development [ 47 ] , drug development [ 1 ] , personalized medicine [ 48 ] , and patient monitoring and care [ 49 ] , among others. As the quality of care offered for patients continues to grow in prominence, here are some ways pharmacies can leverage the continued technology explosion to impact value-based outcomes. As the most accessible and affordable healthcare stakeholder, pharmacies can become health management centers instead of only medication fulfillment locations. Technology can help provide more personalized healthcare offerings including advice, guidance, and an expanded suite of services (e.g., immunizations, screenings, MTM, disease state management). Health trackers and wearable will be able to provide real-time capture of data that can enable pharmacy to follow up with at-risk patients on their conditions and monitor their quality of improvement [ 50 ] .
AI can be of real help in analyzing data and presenting results that would support decision making, saving human effort, time, and money, and thus helps save lives. Medical and technological advancements that have helped the healthcare-related development of AI include the overall evolution of computers, resulting in faster data collection and more powerful data processing, Growth in the availability of health-related data from personal and healthcare-related devices and records, and the development of pharmacogenomics and gene databases, Expansion and industry adoption of electronic health records and natural language processing and other advancements in computing that have enabled machines to replicate human certain processes [ 51 ] .
In the physician space, AI from technology companies like Microsoft is breaking into the healthcare industry by assisting doctors in finding the right treatments among the many options for cancer. Capturing data from various databases relating to the condition, AI is helping physicians identify and choose the right drugs for the right patients [ 52 , 53 ] . In the pharma space, AI is working with researchers to support the decision-making processes for existing drugs and expanded treatments for other conditions, as well as expediting the clinical trials process by finding the right patients from several data sources [ 1 , 54 , 55] . Pharma is even working to predict with certain accuracy when and where epidemic outbreaks might occur, using AI learning based on a history of previous outbreaks and other media sources.
In the hospital space, AI is being used to prevent medical errors and reduce hospital readmissions. By analyzing patient data from medical and medication errors, readmission root causes, and other internal and external databases, AI will one day identify and prevent high-risk patients from developing complications, and provide prospective care guidance, and diagnostic support, among many other clinical applications. Additionally, AI will be useful in workflow optimization and efficiency, helping eliminate redundancy in cost from duplicate or unnecessary procedures [ 56 , 57 ] .
In pharmacy today, we already have an early form of AI in place. It’s called our pharmacy management system, housing patient utilization, and drug data, as well as potentially identifying drug-related problems through clinical decision support screening. The next generation in pharmacy technology is the introduction of a technology-based information expert system to identify timely drug-related problems based on patient data captured from the pharmacy system and other external data systems. Consistent with workflow robotics, this would leave less of the work on the pharmacist to shoulder the responsibility of identifying serious drug-related problems [ 58 , 59 ] .
Implications for pharmacists and their practice
AI can strongly influence and shift pharmacists’ focus from the dispensing of medications toward providing a broader range of patient-care services. The pharmacist can leverage AI to help people get the most from their medicines and keep them healthier. Most importantly, AI provides pharmacy an opportunity for more collaboration across many different entities serving the same patient. For the patient, in addition to potentially better healthcare services offered by their professionals, AI may be a useful tool for providing guidance on how and where to obtain the most cost-effective healthcare and how best to communicate with healthcare professionals; optimizing the value of data from wearable; providing everyday lifestyle guidance; integrating diet and exercise; and supporting treatment compliance and adherence.
Concluding comments
AI involves the combination of human knowledge and resources with Artificial Intelligence. As research into AI continues, with many interesting applications of it in progress, one may consider it a necessary evil even for those that see it as an enemy. Therefore, it is strongly recommended that pharmacists should acquire the relevant hard skills that promote AI augmentation. Education about and exposure to AI is necessary throughout all domains of pharmacy practice. Pharmacy students should be introduced to the essentials of data science and fundamentals of AI through a health informatics curriculum during their PharmD education. Pharmacists must also be allowed to develop an understanding of AI through continuing education. Data science courses or pharmacy residencies with a focus on AI topics should be made available for pharmacists seeking more hands-on involvement in AI development, governance, and use. As these technologies rapidly evolve, the pharmacy education system must remain agile to ensure our profession is equipped to steward these transformations of care.
The opinions expressed in this paper are those of the authors.
Ethics approval and consent to participate: Not applicable.
Consent for publication: All authors approved the manuscript.
Availability of data and materials: Not applicable
Competing interests: All authors declare no competing interests
Funding: None
Author contributions: All authors listed have made a substantial and intellectual contribution to the work and approved it for publication.
Authors’ information:
Muhammad Ahmer Raza, PharmD (The University of Faisalabad, Pakistan), MS Clinical Pharmacy (Shandong University, Jinan, China) is a registered pharmacist (RPh) in Pakistan and an academic pharmacist and pharmacy practice researcher. Shireen Aziz, PharmD (Pakistan) MS (Zhengzhou University, China), is a registered pharmacist (RPh) in Pakistan and completed her MS in Pharmacology from Zhengzhou University, China. Misbah Noreen, PharmD, MPhil (Pakistan) is a community pharmacist in the chain pharmacy setup of Pakistan (Care Pharmacy). Irfan Anjum, PharmD (Pakistan), MS, PhD (Turkey) is an Assistant Professor at The University of Lahore (UOL) Pakistan. Shahid Masood Raza, BPharm (Hons), M.Phil is a lecturer at The University of Faisalabad, Pakistan. He is currently pursuing a PhD from Huazhong University of Science and Technology, Wuhan, Hubei, China. Both are experienced in qualitative and quantitative research methods and content analysis.
List of abbreviations:
MRI Magnetic resonance imaging
CT Computerized tomography
ECHO Echocardiogram
ECG Electrocardiogram
DNA Deoxyribonucleic acid
NHS National health services
GSK GlaxoSmithKline
GNS Gene network sciences
MTM Medication therapy management
Academia.edu no longer supports Internet Explorer.
To browse Academia.edu and the wider internet faster and more securely, please take a few seconds to upgrade your browser .
Enter the email address you signed up with and we'll email you a reset link.
- We're Hiring!
- Help Center
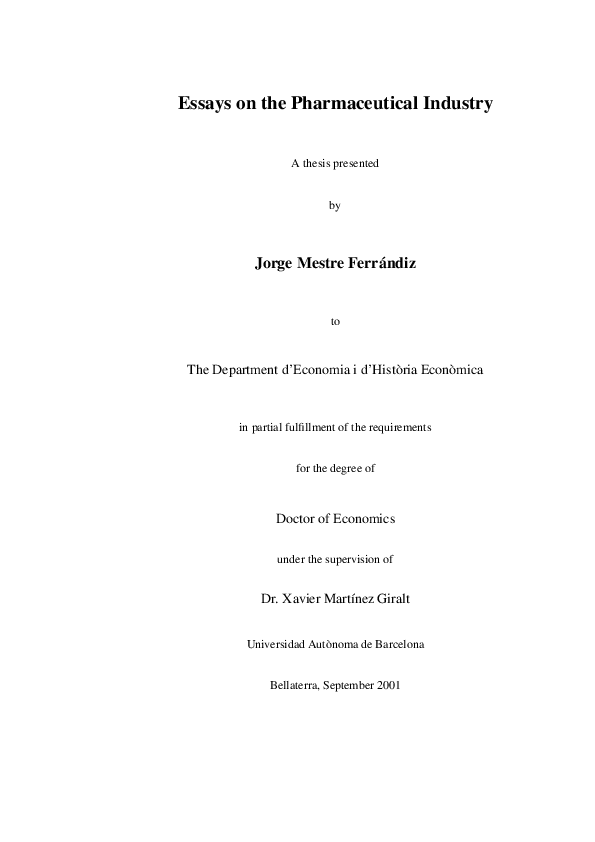
Essays on the Pharmaceutical Industry

Related Papers
Journal of Regulatory Economics
Peter Zweifel
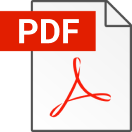
Journal of Health Economics
Odd Straume
Ahmed Abdelhak
Alla Kolyban
The purpose of this study is to test for the existence of moral hazard using a framework of reimbursement policy based on internal reference pricing in Portugal. An econometric model is used to estimate the demand for drugs, employing panel data for the drug market and for different drug reimbursement categories. In general, no evidence of moral hazard is found, but it can potentially appear in two situations. Firstly, moral hazard may occur with the Ministry of Health where the demand for branded drugs includes the most highly reimbursed drugs. Secondly, moral hazard relatively to patients may happen when demanding branded drugs. Another relevant result is the importance of the out-of-pocket difference between generic and branded drugs in determining the demand for drugs. These results are relevant for policy makers in order to improve the implementation and design of reimbursement policy.
Bulletin of the World Health Organization
Dennis Ross-Degnan
William Crown
Lan Vy Nguyễn
Academia Letters
Horst Treiblmaier
Perichoresis
Donald Fairbairn
This article examines Athanasius’ argument in his work Contra Arianos, focusing on the reasons for the order in which he addresses the biblical texts he considers. While the choice of which texts to discuss is dictated by the need to consider those texts that were evidently important in the Arians’ own exegetical arguments, the order in which Athanasius discusses them derives from his desire to begin with biblical texts that clearly describe the whole sweep of biblical redemption. Texts such as Philippians 2:5-11 and Hebrews 1-2 describe in some detail the movements of humiliation and exaltation which the Son undergoes as he becomes man, and thus these texts demonstrate the need to apply any given assertion about the Son either to his eternal existence as God or to his temporal existence as man. In such texts, the literary context-the subject of the passage itself-explicitly describes the broader redemptive context. As a result, these texts constitute the starting point from which t...
maribel antonieta fernandez bejarano
RELATED PAPERS
Hafeezullah Sarparra
U of T多伦多大学毕业证书办理成绩单购买 办毕业证多伦多大学文凭学位证书在读证明
george Rodríguez
Journal of Plantation Crops
Swapan Tripathy
Sakarya University Journal of Education
Rabia Gürbüz
The Scientific World JOURNAL
Sara Bartra
American Journal of Obstetrics and Gynecology
Ricardo Gomez
Monthly Notices of the Royal Astronomical Society
Iranderly F Fernandes
Proceedings of the National Academy of Sciences
Karla Castellanos
Online Submission
Journal of Environment and Earth Science
Dr Jokastah Wanzuu Kalungu
Diccionario del peronismo, 1955-1969 (5ta entrega)
Carlos Ignacio Custer
Muthukumaran Packirisamy
Pi-Chen Chang
Hapidah 261001
Ana Sala Espiell
Revista de Filología de la Universidad de …
Carmen Ruiz
Maj Hasager
rob lichtveld
- We're Hiring!
- Help Center
- Find new research papers in:
- Health Sciences
- Earth Sciences
- Cognitive Science
- Mathematics
- Computer Science
- Academia ©2024
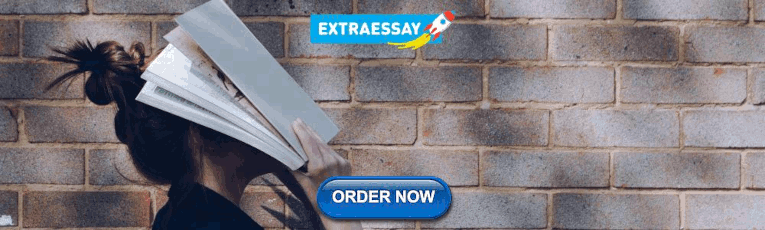
IMAGES
VIDEO
COMMENTS
The total number of pharmaceutical bills by 2007 was estimated to be USD 650 billion among the OECD countries accounting to 15 percent of the health spending. United States is the leading country in pharmaceutical expenditures per capita of $7,960 followed by Norway and Switzerland. Other leading countries include Netherlands, Luxembourg ...
Pharmaceutical Industry the Purpose of. This relationship has an effect on the payment rates that CMS sets. Higher cost pharmaceutical therapies are systematically reimbursed below acquisition cost (i.e., the payment system is biased against full reimbursement for higher cost therapies). Reimbursement compared to acquisition cost for the top IO ...
Torreya estimated the pharmaceutical industry to have a market valuation of US$7.03 trillion by February 2021 from which US$6.1 trillion is the value of the publicly traded companies. Small Molecules modality had 58.2% of the valuation share down from 84.6% in 2003. ... how ghostwriters are employed by the drug companies to write papers for ...
We will write a custom essay on your topic. Pharmaceutical firms first emerged in Germany at the end of the 19th century, but soon gained popularity in other areas of the globe, such as England and America. The pharmaceutical industry grew in pace with the growth of medical technology, expanding the range of products available.
The pharmaceutical industry devoted $83 billion to R&D expenditures in 2019. Those expenditures covered a variety of activities, including discovering and testing new drugs, developing incremental innovations such as product extensions, and clinical testing for safety-monitoring or marketing purposes.
Pharmaceutical Industry - Free Essay Examples and Topic Ideas . The pharmaceutical industry is the branch of healthcare that is involved in researching, developing, producing, and marketing drugs and medicines that are used to treat or prevent diseases or medical conditions. This industry plays a vital role in modern healthcare, as it provides ...
(see: left column in table 1). The global pharmaceutical industry's top supplier of goods or services from other sectors is the chemical sector with a share of 17.0 percent, followed by the agricultural sector with a contribution of 13.4 percent. Vice versa, the global pharmaceutical industry's total intermediate output to other sectors, i.e.
The pharmaceutical market is growing every year ( Figure 14.13 ). According to the forecasts of the Intercontinental Marketing Services (IMS), the world pharmaceutical market will grow 5-8% annually through 2014 and sales will reach US$1.1 trillion in 2014 from US$808 billion in 2009. Figure 14.13.
Achieving these four goals will mean Indian pharmaceutical industry can improve its global market share to ~7.0% by 2030 from current market share of 3.6%17 by value. It will also mean Indian pharmaceutical market will break into top 5 markets in the world from its current ranking of 11th market by value.
The pharmaceutical industry's contribution to fostering health security is recognized globally, and national legislation has been created particularly to regulate the sector . Also, a study to analyze the pharmaceutical industry's role in the Nigerian health security found that government legislation needs to enforce ethical standards and ...
The McKinsey Global Institute estimates that AI in the pharmaceutical industry could generate nearly $100 billion annually across the U.S. healthcare system. The use of AI and machine learning enhances decision-making, optimizes innovation, and improves the efficiency of research and clinical trials.
This dissertation, written by Jiangyun Wan, and entitled Essays on Competition in the Pharmaceutical Industry, having been approved in respect to style and intellec-tual content, is referred to you for judgment. We have read this dissertation and recommend that it be approved. Cem Karayalcin. Timothy Page.
Hagner's innovation spurred Philadelphia's emerging drug industry. In the ensuing years, Hagner focused on pharmaceutical production, establishing the first drug mill in the United States at his East Falls location. He moved to Manayunk in 1820 and built a large steam-powered plant in Northern Liberties in 1839.
India is a major exporter of Pharmaceuticals, with over 200+ countries served by Indian pharma exports. India supplies over 50% of Africa's requirement for generics, ~40% of generic demand in the US, and ~25% of all medicine in the UK. India also accounts for ~60% of global vaccine demand and is a leading supplier of DPT, BCG, and Measles ...
Despite the pharmaceutical industry's notable contributions to human progress,includingthedevelopmentof"miracle"drugsfortreatingcancer, AIDS, and heart disease, there is a growing tension between the industry and the public. Government officials, physicians, and social critics have questioned whether the multibillion-dollar industry is ...
The global pharmaceutical industry has faced unprecedented challenges in recent years, with the COVID-19 pandemic serving as a wake-up call for the vulnerabilities within the pharmaceutical supply chain. As the world strives to emerge from the grip of the pandemic and restore activities reminiscent of pre-pandemic times, uncertainties surrounding supply chain stability persist.
Pharmaceutical Industry essays require a range of skills including understanding, interpretation and analysis, planning, research and writing. To write an effective essay on Pharmaceutical Industry, you need to examine the question, understand its focus and needs, obtain information and evidence through research, then build a clear and ...
The pharmaceutical industry in Europe has a great reputation around the world because of the existing track record that emanates from scientific innovation that contributes to discovery of new medicine. This topic was selected for dissertation because of the relevance of the pharmaceutical industry to economic growth of the European countries.
Pharmaceutical Industry : The Largest Pharmaceutical Company Essay. 3. Overview: AstraZeneca is the second largest pharmaceutical company in UK, which has biopharmaceutical business in over 100 countries. In 2015, company's market capitalization is $70.816 billion, and their net income is US$2.826 billion, with a 4.5% market share of US and a ...
Essay On Pharmaceutical Industry. 1197 Words5 Pages. The Pharmaceutical Industry. The pharmaceutical industry is no longer in the business of helping people, the only thing drug company's now care about is how much profit they can make for their executives and their shareholders. They do this by exploiting their medication and extorting money ...
Hence, there is an increase in the size and variety of biomedical data sets involved in drug design and discovery. This factor and many more contributed to the advancement of AI in the pharmaceutical industry. Today, some companies offer software with much relevance in drug design and data processing, as well as in predicting treatment outcomes.
Essays on the Pharmaceutical Industry A thesis presented by Jorge Mestre Ferrándiz to The Department d'Economia i d'Història Econòmica in partial fulfillment of the requirements for the degree of Doctor of Economics under the supervision of Dr. Xavier Martínez Giralt Universidad Autònoma de Barcelona Bellaterra, September 2001 Acknowledgments I want to thank Xavier Martínez for his ...
Accurate weighing results are key for quality control and production processes within the pharmaceutical industry. In the past, the industry has sometimes lacked a sound metrological understanding of weighing, specifically concerning the performance verification of weighing instruments that includes calibration and routine testing activities. New pharmaceutical regulations in conjunction with ...
DOI: 10.1016/j.jclepro.2024.142550 Corpus ID: 269749912; Application of Life Cycle Assessment in the Pharmaceutical Industry: A Critical Review @article{Chen2024ApplicationOL, title={Application of Life Cycle Assessment in the Pharmaceutical Industry: A Critical Review}, author={Zhengyun Chen and Justin Z. Lian and Hengyi Zhu and Jiawei Zhang and Yulong Zhang and Xinyu Xiang and Dechun Huang ...