- Reference Manager
- Simple TEXT file
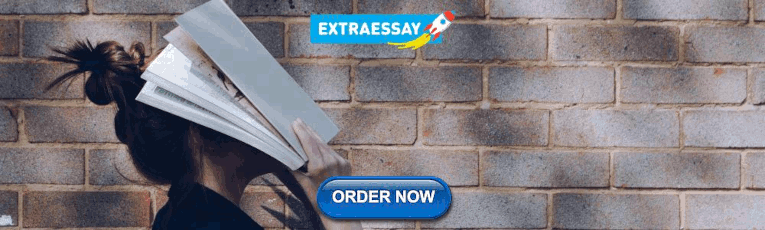
People also looked at
Systematic review article, a critical review of hyperloop (ultra-high speed rail) technology: urban and transport planning, technical, environmental, economic, and human considerations.
- 1 Fachbereich 1, Architektur, Bauingenieurwesen and Geomatik, Frankfurt University of Applied Sciences, Frankfurt am Main, Germany
- 2 Curtin University Sustainability and Policy Institute (CUSP), Curtin University, Perth, WA, Australia
Companies globally have been trying to alter existing transportation systems to maximize public transport benefits, especially in the sector of high-speed (HS) ground transportation. The latest invention of such is Elon Musk's ultra-high-speed rail (UHSR), hyperloop. This technology enables a rail service to operate at a potential speed of 1,200 km/h. Hyperloop's ambitious speed goal has the potential to result in time-space shrinkage, which would then make distant cities more accessible with greatly reduced travel times. Since 2013, several companies have been vigorously promoting hyperloop development in various locations worldwide and competing to construct the world's first functional and commercially viable hyperloop. India, a country struggling with existing public transportation and urban mobility needs, agreed to construct a hyperloop through the company “Virgin Hyperloop One.” This paper first investigates the key technical, environmental, economic and human considerations in assessing the applicability of hyperloop to a particular location. It also considers in some detail the likely urban and regional planning and transport policy implications of the hyperloop technology based on the known effects of existing high-speed rail (HSR) systems. The paper concludes that many of the claims about hyperloop are subject to contrary information, meaning that great caution and prudence are currently needed regarding attempts at commercial deployment.
Introduction
Railways structured urban geographies for most of the 19th and 20th century. They brought prosperity and decline to towns depending on the location of the stations. By the end of the 20th century, the railway's impact on urban form waned considerably due to the proliferation of the automobile and air travel in many parts of the world ( Sideris et al., 2012 ). Therefore, companies have been trying to alter existing public ground transportation systems to maximize public transportation benefits ( Janić, 2018 ). At the beginning of the 21st century, the second railway revolution had emerged called high-speed rail (HSR), operational in numerous parts of the world, including Japan, western and northern Europe, Korea and China ( Sideris et al., 2012 ). In 2013, the CEO of SpaceX, Elon Musk, proposed a concept of ultra-high-speed rail (UHSR) called hyperloop and open-sourced it ( Rajendran and Harper, 2020 ). The technology significantly builds on a much older idea variously known as “gravity vacuum tube,” “gravity vacuum transit,” or “high-speed tube transportation,” which dates originally back to 1865 ( Edwards, 1965 ; Özbek and Çodur, 2021 ).
Since 2013 several companies have been actively promoting hyperloop's development in various locations around the world ( Ravenscroft, 2019 ). Hyperloop is the newest HS ground transport system currently in the early stage of testing. The concept works on electromagnetic attraction forces with travel pods operating in a very low-pressure environment with almost no air resistance or friction, thus enabling the theoretical speed of 1,200 km/h. The technology has an ambitious goal to result in a time-space shrinkage, which will increase the accessibility of cities through very low travel times over long distances ( Musk, 2013 ; Rajendran and Harper, 2020 ). The main selling point used by Musk in his proposal for UHSR is cutting carbon emissions through very high energy efficiency and efficiently managing urban growth. UHSR claims to be superior to other HS transport in operational, economic, environmental and social performance ( Janić, 2018 ). However, due to the hyperloop's very recent nature, its actual effects on cities and people are largely speculative. This paper therefore attempts to investigate the current state of knowledge about the technology and to identify some knowledge gaps in this new mode of transport.
The paper explores the urban and regional planning and transport policy implications of the hyperloop technology as well as its technical, economic, human and environmental dimensions through a wide-ranging literature review. It scrutinizes the validity of the promoters' claims concerning these factors. It discusses the possible development effects of a hyperloop line or network on cities at the regional, urban and station-area levels.
Due to HS ground transportation's relatively recent nature, many variables that influence urban change and spatial restructuring remain largely unmeasured ( Sideris et al., 2012 ). Limitations regarding the availability of information restrict the study within a constrained framework of variables which attempt to cover the physical, technical, economic, environmental, human as well as possible urban planning and transport implications of hyperloop technology. The paper discusses the foundational technical aspects of the hyperloop, but not all the detail. Further, since the hyperloop is a new technology not operational anywhere globally, there are no bases to define its expected behavior; therefore, the paper assumes the hyperloop's expected impacts may be similar in character to that of conventional HSR.
The specific questions the paper seeks to answer are: What are the key technical, environmental, economic and human considerations in assessing the potential application of hyperloop technology in a location? What are its likely urban and regional planning and transport policy implications?
To answer these questions a review of the literature on hyperloop was undertaken to arrive at some consensus about what might be expected from this technology and where caution is due. In the absence of a commercially operational hyperloop, literature on the known impacts of existing HSR on urban and regional planning and transport policy is used to explore the likely effects of hyperloop on those factors.
Literature Review
The word hyperloop was used for the first time in Elon Musk's paper “Hyperloop Alpha” in 2013 ( Musk, 2013 ). However, ultra-HS ground transportation was conceptualized over 100 years ago to achieve fast ground transportation and efficient inter-city travel, gradually evolving over time ( Janzen, 2017 ). There have been various attempts to make this transportation system successful, with hyperloop being the newest invention. Various authors have criticized Musk's work. This review highlights the expected implications of a hyperloop by, and of necessity, drawing on HSR examples worldwide as proxies for hyperloop's possible future urban and regional development and transport policy impacts. It is thus useful at the outset to briefly compare some of hyperloop's claimed characteristics with that of existing and established forms of high speed rail travel, including magnetic levitation rail (maglev). The literature review is then divided into the technical, environmental, economic and human considerations and implications of hyperloop followed by its urban and regional planning and transport policy implications.
Hyperloop Compared to HSR and Maglev—A Brief Overview
To date, HSR, maglev and hyperloop are the only three forms of high speed ground transportation ( van Goeverden et al., 2018 ). HSR and maglevs are commercialized and operate in different parts of the world, the earliest being Japan's Shinkansen line in 1964 between Tokyo and Osaka. Evidence shows that HSR and maglevs have improved the competitiveness of rail against other modes of transport through their dedicated guideways and very fast operating speeds. Additionally, they do not suffer the capacity constraints faced by road-based personal vehicles, they require less energy and produce a lower amount of carbon emission than both cars and planes ( Janić, 2018 ).
By the end of 2011, 17,166 km of HSR was already operational globally ( Garmendia et al., 2012 ). HSR generally connects population centers that are 100 km or more apart ( Rodrigue, 2013 ). Maglevs are also commercially operational, but not at the same level as HSR ( Janić, 2018 ). Currently, just six maglevs are operational in China, Japan, and South Korea and are the highest speed systems available (600 km/h). The three forms of HSR differ from one another partly based on their level of development and maturity ( Ch2m, 2018 ). Table 1 provides a brief comparison between hyperloop, HSR and maglev technologies to show some of their current similarities and differences, which are then further developed and expanded.
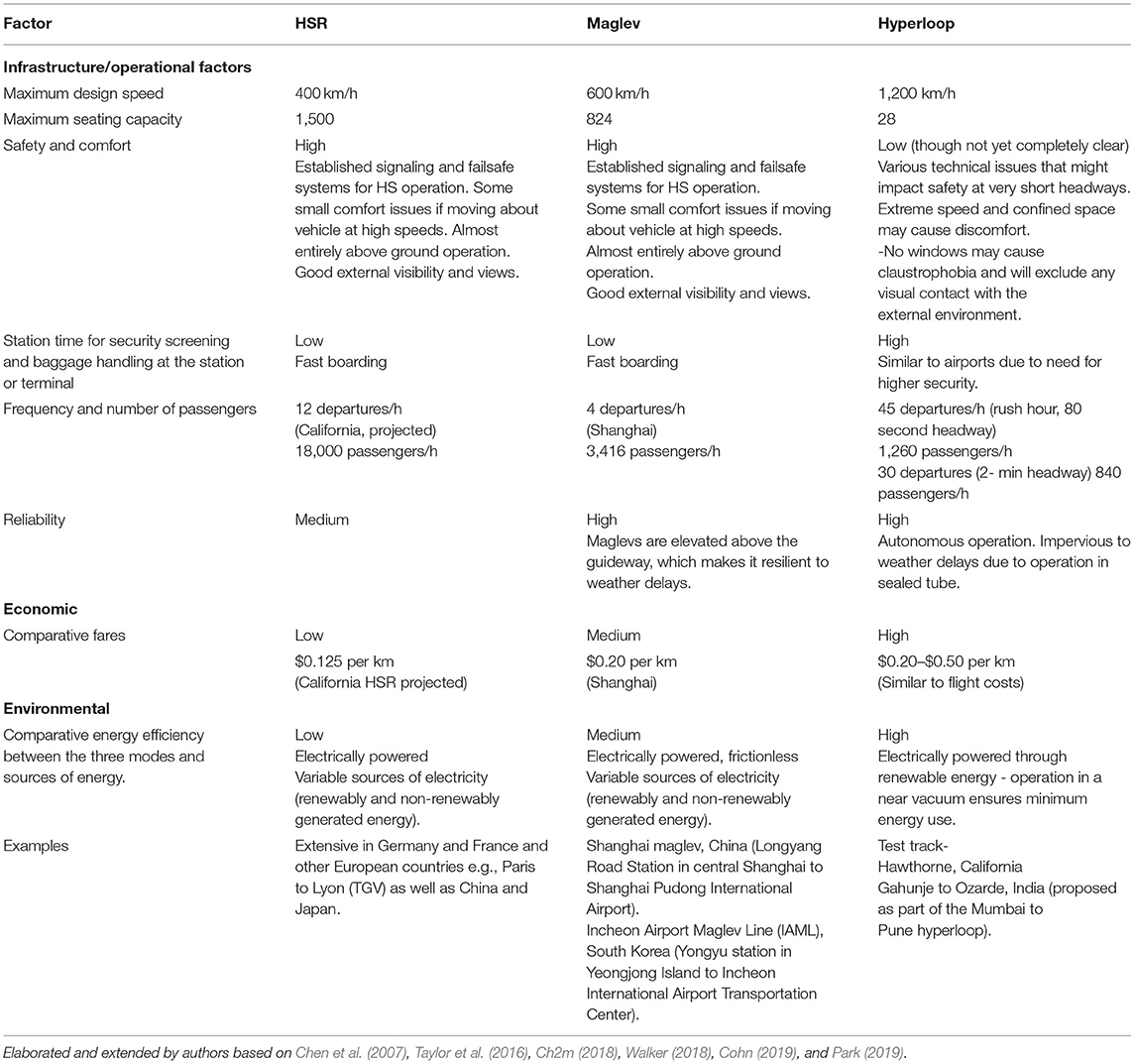
Table 1 . Comparison of hyperloop with HSR and maglev.
The following sections describe in more detail the characteristics of hyperloop and where relevant, its comparison to existing forms of HSR.
Technical, Environmental, Economic and Human Considerations of Hyperloop
Criteria for the selection of hyperloop corridor.
For hyperloop to be successful, it requires certain pre-conditions to achieve its proposed benefits. Musk specified in his proposal that the hyperloop is an ideal solution only for specific cases ( Musk, 2013 ). In their papers, Taylor et al. (2016) and WSDOT (2018) shortlisted six criteria expected to lead to a successful corridor selection for a hyperloop in any country—distance, size, transit connections, economic productivity, congestion and megaregion. These criteria are briefly explained below.
Megaregion, Distance and Size
The hyperloop should connect two important growing cities of the same megaregion. This will ensure selecting cities which are not too far apart. According to Taylor et al. (2016) , with a potential speed of 1,200 km/h, the hyperloop will benefit cities that are at least 500–800 km apart. For trips shorter than 500 km, the net saving time over the automobile will be less due to the check-in procedure, security scanning, and time taken to access hyperloop terminals. Whereas, for station-to-station trips >800 km the cost-effectiveness advantage over aviation will gradually decline.
Economic Productivity, Transit Connection and Congestion
Cities lying at the end of the corridor should have high economic productivity. Also, their intercity connection should be one of the most important in the country and should consist of minimal interconnections with inefficient transportation modes. This will ensure a dense corridor that comprises a high passenger flow, ensuring the maximum potential utilization of the hyperloop benefits after becoming operational ( Taylor et al., 2016 ; WSDOT, 2018 ).
Additionally, according to Walker (2018) , for the hyperloop to operate at its full potential speed (1,200 km/h), the corridor must have flat landscapes. The hyperloop is also expected to be more successful in countries offering political and economic support as there are still uncertainties in its outcome and costs that probably need to be under-written by governments.
Corridor and Terminals
Hyperloop is a guided transportation mode with a specific infrastructure along the route it covers ( Pérez, 2019 ). Therefore, locating the route close to any existing transport infrastructure can minimize the total amount of land required for the new hyperloop infrastructure. Land used to facilitate transport systems above the ground creates opportunity costs as the area cannot be used for other purposes. The hyperloop corridor would ideally be elevated on pillars 30 m apart to limit the land occupation on the ground. However, the narrow space of 30 m between each pillar may remain unutilized. The effective land occupation on the ground (net area) for a hyperloop system's line is estimated to be 0.5 ha/km ( van Goeverden et al., 2018 ). Nevertheless, due to the speed at which hyperloop operates, it has lower flexibility, such that sharp turns are challenging to incorporate, unlike with metro systems or other rail. Therefore, to compensate, the system may use a much larger radius, increasing the required length of guideway, land area and overall cost ( van Goeverden et al., 2018 ).
As indicated previously, hyperloops are currently not commercially operational anywhere, so conventional HSR must be used to speculate on its plausible land-use impacts. Available evidence shows a rapid change in the land use pattern due to the HSR. Zhang et al. (2019) determine the impact of HSR on urban land use in two aspects.
1. HSR stations and routes will cause a direct effect on land use by often consuming arable land. Zhang et al. (2019) analysis of land use transformation in China showed a negative effect on the absolute size of agricultural land in HSR cities due to urban sprawl caused by the HSR. Contrariwise, there was an increase in the amount of new agricultural land in non-HSR cities, offsetting the losses in HSR cities.
2. There will be an extended effect caused by the new transport system's impact on urban development because hyperloop may accelerate urbanization by acting as a catalyst. Various examples of this can be seen globally, such as in Madrid (Atocha Station) ( Zhang et al., 2019 ), Amsterdam (Amsterdam Zuidas), Brussels (Midi) in Belgium and London (King's Cross St Pancreas) in England, where HSR has had a positive impact on land cover rates ( Yin et al., 2015 ).
By extension, hyperloop may accelerate urban development, which might promote urban sprawl and changes in development strategies. It may also lead to the reconstruction of the urban hierarchy which could affect land use policy implementation ( Zhang et al., 2019 ).
Hyperloop is like rail travel in its general appearance and how passengers use it for boarding, traveling and disembarking ( Pérez, 2019 ). It would consist of terminals like at airports with dedicated stations for hyperloop. A HSR station's function is divided into two perspectives according to the node-place model ( Zhang et al., 2019 ), which explains and analyses the station area using a conceptual framework ( Yin et al., 2015 ). As with the node-place model of HSR, as a node , the hyperloop station is expected to connect the catchment area to the hyperloop network. On the contrary, as a place , the hyperloop station may be regarded as a new gateway to the city, increasing the catchment area's status and accelerating land-use changes. This may enable the city to relocate the gravity of the city centre, creating more centres or a polycentric city ( Kenworthy, 2021 ). The hyperloop terminals may lie within the city centre or its outskirts ( Zhang et al., 2019 ) and have different urban impacts depending on their location. Depending on the HSR station's location in a city, Hall (2009) explains three types of urban impacts.
Type 1: It may strengthen a place for commercial investment when the station is located at the city's commercial and business centre. Examples are Chamartín in Madrid, Kings Cross-St Pancras in London and Rotterdam central in the Netherlands.
Type 2: It develops complementary sub-centres for stations situated on the edge of a city. Examples are Kassel Wilhelmshöhe, Germany and Stratford in London.
Type 3: Hall (2009) states the last urban impact as that of developing a new base for the commercial purpose called the “edge city” when the station is located on the urban periphery. Examples are Amsterdam South, Netherlands and Shin Yokohama in Japan.
Type 1 may offer more significant potential for urban revitalization compared to the other two. However, the benefits suggested by existing HSR impacts depend on the current physical and economic circumstances. There are many challenges like competitive dynamics between the old and new station areas and integrating new development into the existing urban structure. Type 2 and 3 effects can spur redevelopment of underutilized areas, giving rise to more urban centres. In return, there will be a connection between more urban centres and nodes within the urban pattern and infrastructure network ( Hall, 2009 ). Similar types of impacts are posited here to occur with hyperloop since there are no commercial systems in place by which to test this conclusively.
Travel Time, Capacity, and Efficiency
The hyperloop's primary value proposition is its energy-efficiency and station-to-station travel time, which would theoretically be faster than other competing modes, flights and transnational rail. However, in its current testing phase, the hyperloop has “only” achieved 400 km/h. Assuming its predicted speed, 1,200 km/h, journey time on hyperloop would be 10–15 times lower than that of conventional rail. This projected time saving has been gaining political and public support ( Walker, 2018 ). Nevertheless, other factors that affect the journey time require consideration.
Transit Time in Terminals
The transit time would take the same as air travel, which would undermine the overall speed advantage of hyperloop, by increasing its overall journey time ( Walker, 2018 ). This is because the hyperloop could be vulnerable to terrorist activity as it is a high-profile asset. To manage the safety risks, there is a requirement for terminal and security screening. The baggage handling process would require special handling staff who would store luggage in separate portions of the hyperloop, like flights ( Taylor et al., 2016 ).
Additional Travel Time to Terminals
The additional time for travel to the terminal adds to the total journey time. Terminals within the city centre will be much easier to access due to their central location and existing access options such as other rail-based modes nearby. The terminals situated in the outskirts might be more of a challenge to access, especially if the terminals are not integrated with other public transport, especially metros or other forms of urban or regional rail ( Walker, 2018 ).
Musk (2013) states that the checking-in process will impact the overall travel less than flights due to the higher frequency between pods' departures. The hyperloop will, ideally, run for 19 h and use 5 h for maintenance ( Pérez, 2019 ). A single pod can hold up to 28 passengers and meet the demand of 840 passengers (off-peak) and 3,360 passengers during peak hours. Walker (2018) criticizes this and argues that this means the pods would have a headway of 30 s during peak hours (or 120 departures per hour), which is questionable due to safety reasons at such high speed. He argues that there should be a minimum gap of 80 s between the pods, allowing 45 departures per hour or 1,260 passengers per hour (only 37.5% of the theoretical capacity).
Other technical problems arise when detailed calculations are performed based on testing the claimed technical characteristics of hyperloop. For example, a 600-km tube would expand and contract by 300 m, taking the California desert with a 40C temperature change between day and night as the test case. This is highly problematic for a system that relies on close to a vacuum for operation (personal communication, Eric Bruun, 12.11.21). Additionally, Bruun calculates and concludes the following:
“If there are only two “tracks” where the vehicles cross over every second run, then minimum headway must be 2 min and capacity is 840–1,200 spaces per hour.” (personal communication, Eric Bruun, 12.11.21). He further concludes that a headway of 40 s is not possible even with a lower safety regime and that a minimum of 60 s headway with no additional margin of safety for braking and acceleration degradation, could result in a collision at +160 km/h speed. And finally, he concludes that even this would require a large terminal with 6 parallel loading tracks. With a realistic two-track terminal, the hyperloop capacity would replace about four wide-body planes per hour, but with no intermediate stops as a bonus, he asks, can billions of dollars in investment costs be justified?
Energy Consumption and Emissions of Greenhouse Gases
Musk's (2013) proposal is a low energy design. This is mainly due to its very low-pressure inner tube design, enabling it to have negligible air resistance and near zero friction due to magnetic levitation or “maglev” ( van Goeverden et al., 2018 ). According to preliminary estimates, the hyperloop is expected to be 2 to 3 times more energy-efficient than its counterpart, HSR. Musk (2013) underlines that the technology will be self-sustaining and cost-effective due to a low energy demand system powered by solar energy. Solar panels mounted on top of the tube will provide the electrical energy which will propel the hyperloop. Based on the research claims, hyperloop may be able to generate more energy than required to operate. Its energy may be stored and utilized to operate the system in situations such as cloudy weather, at night or in tunnels ( van Goeverden et al., 2018 ). However, other research findings suggest that the way to power the system might differ depending on system requirements and terrains ( Walker, 2018 ).
Based on the above facts, more energy would be generated than required for operating the system in places where there is an abundance of sunlight, like Los Angeles and San Francisco or other high insolation locations such as the Middle East ( Musk, 2013 ). Nevertheless, it is not possible to generate solar energy in every country due to climate or altitude limitations. This subverts the potential of the hyperloop's energy performance, so that it may be more efficient and cheaper to generate renewable energy such as solar, wind, water etc., on a large scale depending on region, altitude and climate, which could further be used to power hyperloop via the electrical grid ( Walker, 2018 ; Pérez, 2019 ). Although the emissions of GHGs may be less during hyperloop operations, the indirect emissions may be high due to embodied energy, including constructing the hyperloop corridor, rolling stock and other equipment ( van Goeverden et al., 2018 ).
Construction and Operational Costs
Flyvbjerg (2005 , p. 18) states that it is not necessarily the best mega project that gets constructed “but those projects for which proponents best succeed in conjuring a fantasy world of underestimated costs, overestimated revenues, undervalued environmental impacts and overvalued regional development effects.” One of Musk (2013) proposal's key selling points is its low cost of construction and operation, which would be sourced from public funding ( van Goeverden et al., 2018 ). The construction cost will, however, vary significantly depending on the geographical location, local conditions and engineering challenges ( Walker, 2018 ). Construction in an urbanized area, through mountains or requirement of tunneling would be more expensive than in an empty area of flat sandy soil ( van Goeverden et al., 2018 ). Musk's (2013) proposal shows the construction cost between Los Angeles and San Francisco at $10 million (USD) per km. However, other research companies and media show higher cost estimates of hyperloop ( Mclean, 2016 ; Walker, 2018 ).
Virgin Hyperloop One (VHO) estimates the minimum cost of construction along the same route to be $75.6 million (USD) per km, or 7.5 times more ( Walker, 2018 ). VHO estimated the route from Abu Dhabi to Dubai and Helsinki to Stockholm to be $32.5 million (USD) ( Arabian Business, 2016 ) and $40 million (USD) per km, respectively ( Upbin, 2016 ). However, Walker (2018) further argues that a more realistic value of construction cost would be an increase of 32.3% from the VHO calculations. According to that, the cost of constructing a hyperloop from Los Angeles to San Francisco would be a minimum of $100 million (USD) per km. Ninety-eight per cent of megaprojects constructed around the world suffer overrunning costs. Most rail projects have shown a cost overrun of 50% or higher according to Flyvbjerg (2005) .
Table 2 shows an increase in the value of costs after adding Walker's (2018) assumed realistic value of 32.3% to the costs calculated by VHO. Further, the value increased more after adding the minimum per cent of overrunning costs (50%) for railways to Walker's (2018) calculations.
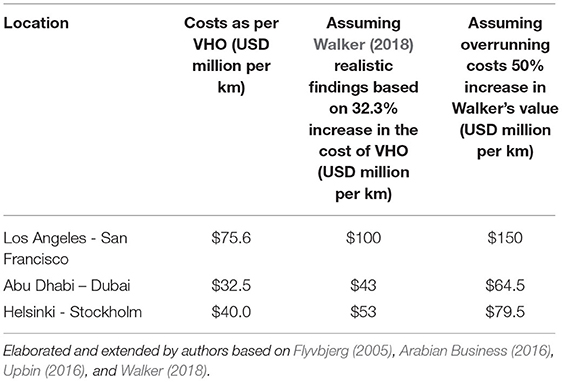
Table 2 . Capital costs of hyperloop in various corridors using different assumptions.
Table 2 shows a significant difference in costs from Musk's (2013) proposal compared to the cost with the addition of Flyvbjerg's (2005) minimum amount of overrunning capital costs. This suggests that Musk's and VHO's calculations are optimistic. Many project proponents believe low costs and high benefit forecasts fit politicians, stakeholders and the media, which allow the project to be realized by sheer momentum ( Flyvbjerg, 2005 ; Zidane et al., 2013 ). One can ultimately only speculate on the actual real-life cost of this megaproject, which, are likely underestimated and therefore will probably cost significantly more than the minimum anticipated cost.
Contrary to Musk's (2013) findings, hyperloop may also not be self-sufficient in an operational cost sense, as not every corridor will produce more energy than utilized; therefore, the highest part of the operational costs (assuming it is driverless), may not be covered. Musk's proposal overlooked some main and mostly operating and maintenance costs that need to also be considered in a full commercial analysis: management planning, infrastructure inspection, insurance, license, infrastructure maintenance (ongoing investment cost), staff, station operating costs (e.g., station labor costs, utilities and water for restrooms, customer amenities, baggage handling, security, connection to other ground transportation, system control, and daily management) ( Taylor et al., 2016 ; Walker, 2018 ). Since hyperloop has no commercial operation experience, these costs are more difficult to estimate ( Pérez, 2019 ). Therefore, it is clear from the above that the hyperloop's operational costs are expected to be much higher than estimated. Indeed, at present, the only people who seem to benefit from any hyperloop profit include engineers, contractors, bankers, landowners, construction workers, lawyers and developers, as the benefits and affordability of such a technology are questionable for the general population, which is detailed in the following section.
Affordability, Equity and Impact on Lifestyle
According to Musk (2013) , a round trip from Los Angeles to San Francisco on hyperloop would cost $40 (USD), which would be affordable to a large portion of the population. As per his findings, the operating and construction costs would be recovered in 20 years through patronage at this fare. However, based on the previous section's findings, such as a likely overestimated number of passengers during peak hour, underestimated construction costs, labor and maintenance, the fare of $40 (USD) appears unrealistic and understated. The costs are more likely to be similar to flights or higher ( Walker, 2018 ). Therefore, the hyperloop might end up being more expensive than anticipated by its investors, resulting in high break-even fares ( Nikitas et al., 2017 ; van Goeverden et al., 2018 ). That being the case, the hyperloop will be “more applicable for the premium passenger transport market” ( Nikitas et al., 2017 , p. 11). Besides this, politicians with a “monument” complex gain satisfaction, administrators get larger budgets and cities get investments that could otherwise be used to develop other cheaper public mobility infrastructures ( Flyvbjerg, 2005 ). This can lead to the loss of “public goodwill” and political capital ( Sideris et al., 2012 ). The resulting high break-even fares would be affordable only by the rich, which leads to more inequity in society. This would cause penalization of the poor, reproduce social policies that are austere and not address climate change ( Monbiot, 2015 ). Therefore, a particular region's economic conditions and political context must be considered in whether such an investment is appropriate and necessary. More precisely, a less technically and economically demanding and appropriate alternative mode or modes of transport should be carefully considered before committing to hyperloop ( Garmendia et al., 2012 ).
Social equity is an essential objective of any form of transportation and is a significant pillar of sustainable development ( Caulfield et al., 2014 ) and therefore important to evaluate and assess before construction. Hyperloops will attract retail activity and raise the value of nearby property and rent ( Zhang et al., 2019 ). Without necessary countervailing policies, this can produce the negative by-product of gentrification, resulting in displacement of poorer populations in favor of wealthier residents who move in to avail themselves of hyperloop's enhanced accessibility. The nature of the station areas may be transformed into higher income enclaves. Taking HSR station examples, this can be seen in the neighborhoods around Brussels Midi, while several disadvantaged ethnic minorities have been displaced in London's King's Cross neighborhood as they could not bid for space in the new development ( Sideris et al., 2012 ). In developing nations, big megaprojects have underperformed in their social and environmental benefits ( Flyvbjerg, 2005 ). Roy (1999 , 2013) describes these impacts in her books The Cost of Living and The Algebra of Infinite Justice by demonstrating the violence and human suffering frequently involved in such megaprojects.
Safety and Comfort
The design proposed by Musk (2013) indicates hyperloop will be safer compared to other rival transport modes, such as airplanes and trains. However, most researchers have criticized this ( Mclean, 2016 ; van Goeverden et al., 2018 ; Zhang et al., 2019 ). Hyperloop is a fully autonomous system consisting of windowless pods that run inside a thick steel tube with a diameter of 6.6 m ( Mclean, 2016 ) built on pillars or pylons ( Walker, 2018 ). The tube acts as a protective cover for the pods, which blocks any interaction with its immediate environment ( van Goeverden et al., 2018 ). This will protect the pod from adverse weather conditions.
As discussed earlier, the small headway between the pods can cause safety concerns if a single pod fails. The kinetic energy generated at the speed of the hyperloop would be massive. It could generate similar impact to 75–200 kg of trinitrotoluene (TNT), an organic compound used as explosives, which can be life-threatening in case of accidents ( Walker, 2018 ). The above safety concerns should be addressed through further elaboration and testing ( van Goeverden et al., 2018 ).
It is unquestionable and self-evident that passenger comfort is essential for any transport mode ( Oborne, 1978 ; Mclean, 2016 ). Hyperloop passengers are required to be seated during operation. The digital displays in the pods also have different options; they can control the position, temperature, power and color of the passenger chair ( Dorsey, 2018 ). Hyperloop movement at ultra-HS, could cause discomfort and fear even with a straight path's slightest deviation ( Nikitas et al., 2017 ). Huge curves would be required to prevent braking speed, so it does not cause motion sickness. The experience of acceleration and deceleration would be felt during the start and the end of the trip. The passengers may also feel vibration and jostling ( Walker, 2018 ). The passenger's comfort is also dependent on seat comfort, lavatory access, disability access and entertainment service ( Mclean, 2016 ). In this regard, being seated inside windowless pods may cause claustrophobia for the passengers. Companies want to solve this problem by developing digital “live” solutions which attempt to recreate window scenery ( Walker, 2018 ). VHO, in their prototype, replaced the window area with displays loaded with personalized entertainment ( Dorsey, 2018 ). Though it might make the passengers feel at ease, it will decrease the human connection with nature even further ( Walker, 2018 ).
Environment
Musk's (2013) proposal promises a counteracting effect on the increasing automobiles and air travel by purportedly reducing roadway congestion, fuel consumption and GHG emissions. However, these goals are questionable on various grounds. If hyperloop does not capture adequate ridership from other modes of transport, its impact on the above variables will be minimal ( Sideris et al., 2012 ). Further, land clearing, tunneling or demolition of historical buildings could be required to construct a hyperloop, which will impact the local physical environment ( Mclean, 2016 ). Evidence shows that natural land is aggressively reduced and fragmented in HSR cities due to the socio-economic activities that follow its construction ( Zhang et al., 2019 ). Hyperloop may have similar environmental impacts, indirectly harming surrounding agriculture and wildlife ( Sideris et al., 2012 ). Therefore, it is essential to strengthen natural land management in hyperloop-connected cities ( Zhang et al., 2019 ). Ideally, a hyperloop should only be proposed for corridors where it would cause minimal cutting of trees and demolition of historical buildings.
Where the hyperloop station areas are poorly integrated with other modes of public transport, they will attract more private vehicles giving rise to more parking demand. This is especially so when the stations are to be located on the outskirts, which often lack integration with other public transport connections as well as non-motorized modes ( Sideris et al., 2012 ). According to van Goeverden et al. (2018) , the vacuum pumps from the hyperloop may also cause noise. The elevated hyperloop corridor pillars, which are not properly embedded in the urban fabric, may cause visual intrusion. These factors may create a “barrier effect” for the people residing or working in adjacent communities ( Sideris et al., 2012 ). It may cause annoyance and a harmful effect on these people, limiting the possible use of public space. Residents may even prefer to move to other neighborhoods away from the terminal and corridor. Regarding land use, this may lead to development of previous open space, involving problematic opportunity costs ( van Goeverden et al., 2018 ). Therefore, the terminal areas must be planned and integrated well with public transport connections, as well as non-motorized modes.
Urban and Regional Planning and Transport Policy Implications of Hyperloop
Hyperloop spatial development effects at the regional level.
The high speed of hyperloop will allow it to improve intercity accessibility, as suggested by other operational HS ground transportation impacts ( Janić, 2018 ). Through the hyperloop line, where there is an intermediate station every 500–800 km, passengers would be able to travel long distances for different purposes, such as work or tourism in very reduced time ( Musk, 2013 ). This will change the absolute and relative accessibility of cities at the regional level ( Chen et al., 2019 ). Therefore, long-distance traveling by hyperloop at high speed will bring distinct parts of one country together ( Brunello, 2018 ).
Since hyperloop is a relatively new technology, the development experience of HSR, will be used to explain hyperloop's expected impact on urban development behavior. Various researchers have used modal and empirical-based approaches to investigate HSR's regional spatial development effects ( Brotchie, 1991 ; Chen and Hall, 2011 ; Yin et al., 2015 ). They monitored the development change in HSR connected regions with the unconnected ones in Japan ( Brotchie, 1991 ) and the United Kingdom ( Chen and Hall, 2011 ). The result shows a clear relationship between cities connected to HSR and urban development patterns. The connected cities were influenced by HSR through the creation of new location advantages for individuals and firms in terms of private housing, offices and knowledge-intensive activities ( Brotchie, 1991 ; Chen and Hall, 2011 ). In other words, the location factor created more opportunities and high-level commercial activities that attracted more people from faraway places ( Yin et al., 2015 ). Hence, this transformed the spatial structure and expanded the market and labor potential, which in turn improved the connected city's overall performance in Japan and the United Kingdom ( Brotchie, 1991 ; Chen and Hall, 2011 ).
HSR connected cities showed an economic advantage as transport hubs that improve the city's status and competitiveness ( Pol, 2002 ; Chen and Hall, 2011 ; Chen et al., 2019 ). Improved status leads to economic spillover ( Chen and Hall, 2011 ), which enhances spatial competition and causes an increase in land value, resulting in higher real estate rent and cost of living ( Brotchie, 1991 ; Zhang et al., 2019 ). Through this, certain people will move to less expensive nearby unconnected cities triggering their growth. In return, this can attract back-office workers to these unconnected cities ( Zhang et al., 2019 ). Conversely, the unconnected cities also experience several challenges, such as unemployment and reduced accessibility ( Chen and Hall, 2011 ). Hence, there is an imbalance between cities connected to HSR and those that are disconnected ( Pol, 2002 ).
Hyperloop is expected to have similar spatial development impacts along its corridor. Therefore, the new location advantage of cities connected to hyperloop result in considerable disparity with the non-connected ones ( Zhang et al., 2019 ). Also, the unemployment in unconnected cities will accelerate migration to the connected ones, which would in turn restrain the development of peripheral cities ( Hall, 2009 ). This tends to contradict Musk's (2013) statement that hyperloops will make cities less crowded as people will be able to travel further away for work. Rather, the previously mentioned impacts of hyperloop suggested by HSR experience, such as gentrification and unemployment, envisage a mixed picture. Hyperloop seems to facilitate both decentralization and concentration in hyperloop-connected cities.
Musk's proposal states that hyperloop should have a maximum of three or no intermediate stops to access the full potential of its speed ( Musk, 2013 ). The trade-off between accessibility and speed risks concentrating services on profitable or major cities ( Garmendia et al., 2012 ; Brunello, 2018 ). Intermediate cities bypassed in the vicinity of the hyperloop corridor will experience reduced service levels and limited access, which will reduce their ability to generate substantial traffic, causing a marginalization risk for these cities ( Brunello, 2018 ). This can drain the economic activities of intermediate cities, causing an overall negative impact called the “backwash effect” ( Berg and Pol, 1998 ), seen for example in Nagoya, Japan along the Tokyo-Osaka HSR line ( Kamada, 1980 ). In this process, core cities are drawn closer, while distant places tend to get more remote. Hall (2009) refers to this as “peripheralization of the periphery.” These factors are most visible at the regional level. This is because different parts of the same region experiencing various levels of accessibility are measured, thus exacerbating their relations ( Brunello, 2018 ). However, according to Chen and Hall (2011) , these challenges can be avoided by integrating unconnected cities with connected ones through other modes of transport, such as metro or other conventional rail systems. This will improve inter-regional and intra-regional accessibility.
It is clear from the above evidence, that hyperloop can reconstruct the political and regional economic structure by redistributing socio-economic activities ( Zhang et al., 2019 ). However, it is essential to know if the development opportunity would differ based on the type of city connected to the hyperloop, again drawing on HSR experiences. This can be summarized as follow.
Major Cities (First-Tier Cities, International Service Cities)
First-tier cities are already dominant cities; connection to HSR will enlarge their area of influence and they would tend to become even more competitive ( Cervero and Bernick, 1996 ; Moyano et al., 2019 ).
Intermediate Cities (Second and Third-Tier Cities, Cities in Transition)
According to Cervero and Bernick (1996) , intermediate cities connected to HSR have a more significant development impact than the major cities. Second tier and third-tier cities can overcome isolation, improve their location advantages and attract business activities. They will also significantly attract and increase tourism ( Zhang et al., 2019 ).
Pol (2002) and Chen et al. (2019) agree and state that HSR improved the primacy for “international service cities” and visibility of “cities in transition” ( Sideris et al., 2012 ). Hence, cities show a generative economic growth. However, Hall (2009 , p. 68) argues that HSR “will be the maker of some cities but the breaker of others.” The catalyzing or facilitating role of cities due to connection to HSR brings changes that lead to improving their position in the urban hierarchy. However, this could also lead to unwanted side effects for cities already existing higher in the national and continental hierarchy, such as loss of activities. Instead, this causes the redistribution of the economy. Therefore, cities need to meet preconditions for economic growth and renewal to achieve positive economic growth ( Pol, 2002 ).
As suggested by HSR, the development opportunity and growth associated with hyperloop are expected to differ similarly based on the type of city, major or intermediate, connected to the hyperloop. Hyperloop-connected major cities may become more competitive, whereas intermediate cities may attract more tourists. However, this catalyzing role of these cities connected due to hyperloop will redistribute the economic activity of regions.
To examine the expected regional structural effects of hyperloop it is important to take the results of before and after development effects of cities connected to HSR to provide the anticipated hyperloop behavior. Garmendia et al. (2008) and Preston and Wall (2008) have compared this in Ciudad in Spain and Ashford in England. Their findings show that HSR can aid isolated, intermediate cities to attract housing investment from within the region. Post-HSR connection, both cities increased their spatial polarizing capacity and increased their population. HSR facilitated the development of small cities as specialized sub-centers of metropolitan areas with high-level office development. That way, people do not need to commute to the central or inner areas of the metropolis ( Yin et al., 2015 ). Hyperloop can most likely be expected to facilitate similar development changes as HSR.
Indirect Effects of Hyperloop at the Urban Level
Hyperloop terminals and urban development should reinforce each other. Their terminals must be developed in synergy with urban dynamics with “cities in transition” and “international service cities.” This section discusses the development of hyperloop in synergy with urban dynamics, using HSR impacts as a general guide to expected hyperloop impacts.
Muller (2004) findings show that London's and Paris's urban development patterns were more noticeable after the construction of their metro systems. The connection between the city centre and its periphery improved. Hence, transport infrastructure and urban development are largely path dependent. Similarly, the urban development patterns in hyperloop-connected cities will help determine the conditions for its performance, good or bad. The synergy between HSR and urban dynamics is shown below in Lille, France and Amsterdam, The Netherlands.
HSR Attracted by Declining Regions
The city of Lille was an industrial centre that also included a university and a traditional historical centre. In the 1960s, due to the industrial downturn, there was high unemployment and an increasing gap between social and spatial segregation. When the plan for HSR was drawn up, the rail operators were skeptical of using Lille as an intermediate stop between Paris and Brussels due to the expenses, potential financial loss, less direct route and loss of time. However, the construction of HSR in the centre of Lille proved to be successful. The station was constructed in a manner complementing the existing centre to maintain balance and avoid competition. It led to the revitalization of the city centre, which improved its economy. The station gained new facilities, attracted offices, commercial areas, cultural establishments, public facilities, housing and public spaces ( Bertolini, 2000 ). Therefore, HSR benefited from Lille's economy by reinforcing Lille's regional economic activities and its commercial centre.
HSR Attracted by Active, Lively Stations
In 1990, Amsterdam experienced an increase in population growth due to an increase in the global economy. The growth led to the requirement of new economic spaces. Therefore, banks, business and residential developments started to move to the edge of the city ( Salet and Majoor, 2005 ). Due to these changes, Amsterdam Zuidas (the southern part of Amsterdam), became the new focal point. In 1997, plans were drawn to construct a HSR station at Zuidas. However, the operators feared that connecting Zuidas would elevate its area in the urban hierarchy and create an imbalance with the rest of the city ( Bruinsma, 2009 ). After Amsterdam Zuidas' connection to HSR, it encouraged balanced development throughout the city. People saw the need to change the monocentric structure of the city and adopt the multi-nuclei (polycentric) model, which led to high quality, mixed-use development throughout the city ( Salet and Majoor, 2005 ).
As seen from the above, these two cities achieved synergy between HSR and their own urban dynamics and witnessed a positive development impact. Similarly, hyperloop connected cities, with and without advantages, may reinforce urban development and growth on an urban level. However, there are various challenges involved.
Hyperloop may cause rapid urbanization that will accelerate the rate of urban expansion and decrease cultivated land ( Zhang et al., 2019 ). According to Brenner (1999) , a new transport system is a significant driver in influencing urban form. Evidence of other operational modes of HS ground transportation shows the rapid change in land use patterns due to station development or redevelopment. Studies also show a connection between the land value of housing property and railway infrastructure. For example, the areas within a walking distance of 10 min to the station (about 800 meters) have distinctly higher land value due to the attractiveness of retail activity in the area which in turn is driven by the improved mostly pedestrian-based “movement economy.” On the other hand, certain factors can bring down value, such as noise and crime effects often associated with railway stations. However, the impact of the first may be more significant than the latter ( Yin et al., 2015 ; Zhang et al., 2019 ). An example of this can be seen in the Frankfurt central station area, Germany ( Möbert, 2018 ; FAZ, 2020 ). Flyvbjerg (2012) agrees and further states that urban megaprojects are usually poorly integrated with the rest of the city's planning. Only certain parts of the city, mainly the areas around the station, receive special planning. Therefore, the build-out of space by hyperloop will not be uniform throughout the city. It will create an imbalance between cities and their hinterlands. The serviced areas will improve their status, modernity and value ( Chen et al., 2019 ). The result will tend to be gentrification around the station area, causing displacement of lower income populations and an influx of higher income people ( Sideris et al., 2012 ). Therefore, hyperloop may facilitate polarization and segregation between station areas and other parts of the city. The differential increase in accessibility accompanied by hyperloop may lead to spatial inequality and centralization ( Chen et al., 2019 ).
According to Musk (2013) , hyperloop will mitigate the relocation of household and economic activities from moving outwards within a city, i.e., less urban sprawl. However, as suggested above, hyperloop might cause gentrification which may further encourage sprawl, since many people may move toward the periphery where land is more affordable compared to station areas. Further, the potentially rapid and extensive development in cities due to hyperloop, may cause various challenges, termed broadly as the “urban disease.” These include weakly built physical environments, loss of urban and environmental quality and traffic congestion ( Sideris et al., 2012 ).
Micro Effects of Stations on the Urban Development Patterns of Adjusting Neighborhoods
HS ground transportation contributes to the identity of the surrounding area. Their stations' functions are like that of a traditional railway station area ( Yin et al., 2015 ). Zhong et al. (2014) defines the station area as the development perimeter and the zone of influence based on accessibility levels. It is vital for a city and its inhabitants to link the station's catchment area with the transport network ( Zemp et al., 2011 ). The catchment area for a HSR terminal is much larger than that of traditional rail stations. Its catchment area falls in the radius range of 5–40 km, depending on the feeder system ( Zhong et al., 2014 ). As various researchers have used HS ground transportation's catchment area as the minimum of 5 km ( Murakami and Cervero, 2010 ; Yin et al., 2015 ), this research assumes the same. The hyperloop can be reached within 15 min by using typical complementary transport modes such as trams, metro, suburban rail and buses with an average speed of 20 km/h, a typical urban bus speed average speed ( Kenworthy and Laube, 2001 ). However, the hyperloop station's accessibility in the immediate station area catchment of 5 km and distances higher than 5 km would generate different development effects in these areas. As mentioned earlier, there are no operational examples of hyperloop to define these differences clearly. Therefore, HSR is used to suggest these differences. Yin et al. (2015) divided the area around HSR stations into three zones, primary, secondary and tertiary development zones ( Table 3 ).
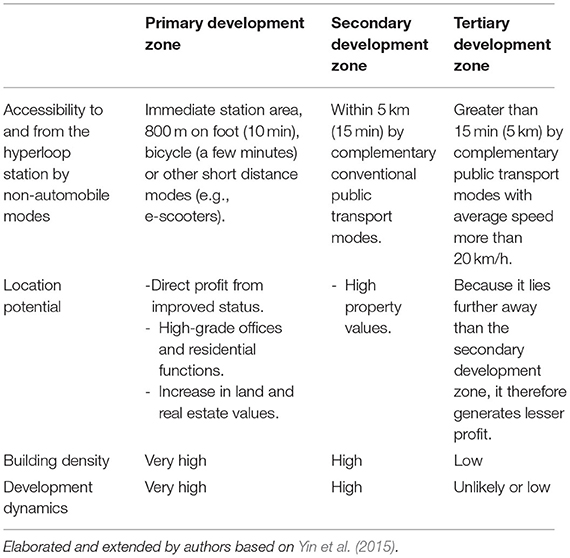
Table 3 . Likely development effects of a hyperloop station based on the respective development zones.
The primary zone witnesses the most significant number and magnitude of effects due to direct contact with the station area. This zone consists of the highest land prices, high-grade offices and residential functions because of its proximity to the station, which improves its status, leading to high levels of dense development. The secondary zone is reachable within 15 min from the station via complementary conventional modes of public transport such as metro, tram, suburban rail, BRT or in many cases urban buses, provided they can operate at an average speed of 20 km/h or more. The development, density of buildings and land value are still high but less compared to the immediate station area. The tertiary zone is less likely to see development due to its furthest proximity to the station ( Yin et al., 2015 ). As suggested by the above HSR impacts, the hyperloop terminal is expected to induce similar development patterns.
Certain preconditions are essential and will influence the intensity of development in the zones mentioned above, such as strength of the regional economy, the existing image of the city, integration of the HS transport mode with other modes of transport, location of the station, mixed-use development in the station area and balanced embedding of the new transportation in the existing urban fabric ( Jong, 2007 ; Walker, 2018 ). Good car accessibility in more peripheral locations would also be influential in certain circumstances, but generally in denser or more central locations, non-automobile modes are preferred due to their spatial efficiency (cars require high road and parking capacity near stations) and their ability to deliver large number of potential patrons.
HS ground transportation's effects on long-term spatial development are complex processes, which are rarely isolated and less quantifiable. The development effects include the revitalization and new development of neighborhoods around the station area. Examples can be seen in Amsterdam Zuidas in the Netherlands or Lisbon East in Portugal. These station areas showed an improvement and increase in trade, socio-cultural facilities, new residences, creation of public spaces and the quality of the urban districts through green areas ( Peters, 2009 ; Vale, 2015 ).
Another positive effect is the regeneration and revitalization of formerly derelict areas. An example of such an area is the mall at the HSR station in Euralille, Lille (France). HSR might also aid in defining new city cores and commercial centres, as can be seen at Shin-Osaka in Japan. Some cases of HSR also showed the redevelopment of formerly brownfield sites and railway properties such as Stratford in England. Another visible effect is the combination of convention centres, retail and entertainment facilities in new architectural landmarks and new, attractive and significant buildings such as in Berlin, Germany and Lille, France ( Sideris et al., 2012 ).
It can be concluded that hyperloop may act as a catalyst for new commercial and residential development and facilitate urban regeneration. This would generally involve enormous economic spinoffs. However, these impacts differ depending on context and circumstances. Specific operational modes of HS ground transportation experiences in the past have also shown an opposite impact. For example, Berlin post-HSR showed no signs of new development or redevelopment around its station area. Berlin is Germany's poorest large city with the lowest GDP per capita ( Kenworthy, 2013 ). Other similar examples are Tours station in France, Ashford station in England and the Ebbsfleet International HSR station, also in England. These stations have done little to regenerate the areas around them, which so far mainly witness the building of park-and-ride facilities ( Hall, 2009 ).
In effect, station-adjacent neighborhoods of hyperloop may or may not act as catalysts for desirable patterns of development and growth, as suggested by the diversity of HSR impacts. Therefore, hyperloop may have varied impacts on the local context. However, it is mostly generative, as suggested by most HSR experiences. Any hyperloop service and its associated terminals may cause an increase in government subsidies and opportunity costs because of forgone other services caused by the enormous likely expenditure for hyperloop. The loss of public goodwill then becomes a potential problem and the political cost of foregoing the development of other essential infrastructure, which may be more beneficial to a particular area, is a problem for everyone concerned ( Sideris et al., 2012 ). The hyperloop station area would be expected to have a high population density. A high population or dense area, however, usually confronts institutional barriers in its creation, which can sometimes thwart its fruition ( Taylor et al., 2016 ). There is a risk of urban fragmentation of the station area through developing into a separate “island” in terms of spatial and functional development, ownership, control, scale and architecture ( Trip, 2004 ; Sideris et al., 2012 ). The urban planning and urban design of the station should be done carefully and with maximum public participation to complement its surroundings and mitigate these unfavorable conditions.
Integration Between Hyperloop and Urban Transport Networks
Different modes of transport are elements that contribute to the making of the entire transport system in an urban area. Each element must contribute to bringing its passengers within the access point of other elements for most trips ( Yin et al., 2015 ). The interaction potentials between urban nodes in the transportation network are measured using accessibility, accessibility depending essentially on the proximity between nodes, their relative size or attraction potential and the quality of the transport connections between those nodes, which results in greater or lesser travel “friction” ( Black, 1981 ). Hence, accessibility is of critical importance to the transport network ( Yang et al., 2018 ). Secure integration of hyperloop with other modes of transport will prove to be crucial to its overall performance in terms of its system, passengers and the region served by its lines. This integration will enable hyperloop lines to link with regional services, which could then operate in harmony with the hyperloop through connections and matching timetable. This should ideally avoid disorderly competition, encourage a sensible distribution of occupancy, and stimulate cooperation among operators. More passengers would be able to reach hyperloop stations, increasing its spatial penetration ( Yin et al., 2015 ) while simultaneously enhancing use of sustainable modes of transport connecting to it.
The hyperloop should ideally involve a seamless physical integration between the connection modes and the transfer station ( Sideris et al., 2012 ), the aim of a connector being to reduce “transfer resistance” ( Yin et al., 2015 ). HS ground transportation relies on integration with rail and bus (and non-motorized modes for proximity to stations) to provide a door-to-door service ( Berg and Pol, 1998 ), using timetable matching and integrated ticketing ( Hall, 2009 ), which will also aid in reducing “transfer resistance." On the other hand, weak integration will cause low passenger volume, which indirectly works in favor of cars and in the long term, lowers the development impact on the stations' primary and secondary development zones. This causes delays and connection disruption during rush hours and affects the area's development potential ( Yin et al., 2015 ).
Evidence also shows HS ground transportation location poses challenges for other modes. According to Walker (2018) , locating the hyperloop station in the city center would prove to be more beneficial. This is because hyperloops may be able to provide an opportunity to use the already available infrastructure (metro or other rail) to help develop their stations or terminals ( Sideris et al., 2012 ). This would save terminal costs, travel and waiting time of passengers and ease of integration with other modes of transport ( Taylor et al., 2016 ). For instance, Taiwan started constructing HSR in 2000, which was operational from 2007 ( Kao and Lin, 2007 ). The construction of HSR stations in Taiwan is mostly on the outskirts of urban areas with no integration of public transport, leading to poor performance due to a decline in passenger requirements and timetable matching. Although the government aimed to integrate public transport with the existing HSR stations and strengthen it, it was not easy to achieve ( Cheng, 2010 ) due to technical issues, operational challenges, civil engineering and financial challenges ( Sideris et al., 2012 ). On the other hand, the HSR in Japan was successful due to integration, reinforcement and improvement of public transport services with it, such as narrow-gauge rail lines, bus and ferry ( Okabe, 1980 ).
Locating HS ground transportation in the city centre unfortunately also poses a few challenges. It will have higher costs associated with land acquisition than peripheral locations ( Taylor et al., 2016 ). It might also be a challenge to create additional capacity for a hyperloop station in the already existing transport system located in centres. In these situations, underground construction may be a solution. However, governments tend to rule this out due to high costs ( Yin et al., 2015 ). Therefore, locating a hyperloop station on the periphery is cheaper in construction and land costs but also characterized by poor or low public transport accessibility, and more expensive, though easier car accessibility. City centre locations would prove to be more beneficial through careful planning to avoid the earlier mentioned challenges.
Even though hyperloop would compete with air travel, integration of it with airports will provide a complementary service ( Walker, 2018 ). It could provide fast, easy access to airports and enlarge its service area in terms of intercontinental flights. HSR experience of this has been witnessed by Lyon-Satolas airport in France. There was a massive decline in the volume of passengers initially at the airport after the HSR began in 1981. However, after the construction of the HSR terminal at the existing airport there was improved accessibility to an enlarged radius of 400 km. This, in return, encouraged intercontinental flights. By 1996, Lyon-Satolas surpassed its international traffic mark of 50% ( Yin et al., 2015 ). In the current situation of potentially devastating global climate change, however, increased air travel must also be seriously questioned because of its huge CO 2 emissions and other impacts in the upper atmosphere.
In conclusion to the integration of hyperloop with other transport networks, companies envisage, strong planning of inter-modality. To meet the demand of passengers' needs and transfers, any hyperloop station should provide secure, good access and consistent punctual departures and arrivals through reasonable timetables and suitable places for boarding, disembarking and waiting. The stations should also provide excellent spatial orientation and design for passengers to change between modes. Passengers also require information and related services such as ticketing in waiting areas and beyond ( Zemp et al., 2011 ). A great deal has been posited in this section about the abilities of the hyperloop. In the case of achievement, the hard evidence of success or the best way to proceed is much less clear. Hyperloop proposals need to address issues specific to it and from the experience of other HS ground transportation. Real-world demonstrations would be required to overcome the potential issues related to safety, economics, and passenger comfort ( Walker, 2018 ).
Conclusions
This paper set out to answer two key questions: What are the key technical, environmental, economic and human considerations in assessing the potential application of hyperloop technology in a location? What are its likely urban and regional planning and transport policy implications? Musk's ideal hyperloop is said to reach ultra-high speeds of around 1,200 km/h, though evidence in the literature suggests more likely speeds of around 500 km/h due to the practical realities of engineering in different locations coupled with limits of passenger comfort. It will have a relatively low carrying capacity per departure due to each pod having only 28 seated passengers. Theoretical peak hour capacity of 3,360 passengers depends on 30 s headways which, for safety reasons, may need to be 80 s, reducing peak capacity to 1,260 passengers. Furthermore, evidence suggests that companies have underestimated the costs involved with the system. These and other technical, environmental, and human considerations were questioned throughout the paper and for the most part, the positive claims for hyperloop are disputed or at least suggested to be overstated.
Such problems could lead to loss of public goodwill and political legitimacy on the part of governments seeking to install hyperloop without a very thoroughgoing investigation of its implications for specific corridors and station locations. A key problem appears to be that it will likely only be affordable for travel by higher income populations which can add to an already large societal gap and accelerate further inequity. In human terms, traveling at an ultra-high speed with no windows in the pods detracts from hyperloop's comfort level and acceptability for people, notwithstanding any efforts at virtual entertainment. Additionally, hyperloop's terminals may attract parking lots, visual and noise pollution if not carefully planned to maximize interconnections via conventional public transport, bicycles and walking and with genuine public participation.
Based on diverse experience with conventional HSR, hyperloop may cause barrier effects and limit the use of urban space for communities, in particular green space, if the possible densification and land use change impulses from land value/real estate price increases around terminals are not properly planned with the broader community good in mind. There are also potential impacts on near-city and within-city agriculture, which are discussed in the paper and need to be considered, especially in rapidly industrializing cities where agriculture and other food production in urban hinterlands is still very important. Broadly speaking, the available evidence would tend to suggest that there are more sustainable approaches to regional and urban mobility that need to be thoroughly investigated and comprehensively compared to hyperloop, in environmental, economic and social terms, before decisions are taken to seriously consider hyperloop.
This is especially true when considering the potential urban and regional planning and transport policy implications, which are very diverse, as elaborated in detail in the paper. On a regional, urban and micro level, it is anticipated that hyperloop provides the possibility of broad transformations very like HSR experiences around the world, some of which have been good, some bad and some cases where there has been little effect, depending on the broader economic, political and planning circumstances. Specific cases are explained in the paper. For example, like HSR, hyperloop may bring distinct parts of the city together, yet the imbalance that could be generated between hyperloop-connected and unconnected cities is a critical finding to be examined by authorities and the operating companies. Musk's statement that the hyperloop will make cities less crowded is a double-edged sword. This claim could be true regarding certain aspects while untrue for others, as it would likely facilitate both decentralization and concentration at the same time.
In the likely event that hyperloop would be financed through government subsidies, just like most HSR-projects, opportunity costs are almost certain to occur. This will very likely result in the absorption of capital funds essential for other critical transport infrastructure projects such as BRT, metro or better walking and cycling facilities. This makes it essential to perform a comprehensive comparative analysis of the benefits vs. costs of investment in hyperloop vs. other crucial infrastructure needs in any specific area or transport corridor. Therefore, a wide range of highly skilled and specialized people are required to keep the issues mentioned in this paper in close consideration to legitimately and confidently assess, in any given situation, this so far real-world unproven technology.
Based on the findings of this paper, we conclude that there exist many positive claims about hyperloop, mainly by its proponents, but for each one of these claims there are counter-claims, mainly by experts in the rail field, which cast severe doubt on the viability of hyperloop both as a technology and as a tool in helping to reshape urban regions to reduce the time and space separation between cities. The review of existing literature in this paper has attempted to systematically bring together both sides of the story and to compare the claims of each. Based on this approach, we conclude that the counter-messaging about hyperloop is convincing and therefore warrants a high degree of caution in considering it for deployment anywhere.
The other clear message is that it would be foolhardy for any government to commit to building a commercial hyperloop line or system without much more thorough testing of prototype systems in different conditions. Accordingly, the development and construction costs of any hyperloop in the foreseeable future should be borne to the largest degree by the proponents of the system, not by the governments to whom hyperloop is being marketed. Over-commitment to hyperloop now by any government, especially financially, would not be prudent.
Data Availability Statement
The original contributions presented in the study are included in the article/supplementary material, further inquiries can be directed to the corresponding author/s.
Author Contributions
SP and JK were involved in the writing of the original manuscript and its continual review and improvement. Both authors contributed to the article and approved the submitted version.
Conflict of Interest
The authors declare that the research was conducted in the absence of any commercial or financial relationships that could be construed as a potential conflict of interest.
Publisher's Note
All claims expressed in this article are solely those of the authors and do not necessarily represent those of their affiliated organizations, or those of the publisher, the editors and the reviewers. Any product that may be evaluated in this article, or claim that may be made by its manufacturer, is not guaranteed or endorsed by the publisher.
Arabian Business, (2016). Abu Dhabi-Dubai Hyperloop Route Said to Cost $4.8bn . Available online at: https://www.arabianbusiness.com/abu-dhabi-dubai-hyperloop-route-said-cost-4-8bn-650603.html (accessed January 7, 2020).
Berg, L., and Pol, P. (1998). The European High-Speed Train and Urban Development: Experience in fourteen European and Urban Regions . Ashgate: Aldershot.
Google Scholar
Bertolini, L. (2000). Planning in the borderless city: A conceptualization and an application to the case of station area redevelopment. Town Plan. Rev. 71, 455–475. doi: 10.3828/tpr.71.4.2883q121q1671185
CrossRef Full Text | Google Scholar
Black, J. (1981). Urban Transport Planning: Theory and Practice . London: Routledge Library Editions.
PubMed Abstract | Google Scholar
Brenner, N. (1999). Globalization as reterritorialisation: The re-scaling of urban governance in the European Union. Urban Stud. 36, 431–451. doi: 10.1080/0042098993466
Brotchie, J. (1991). “Fast rail networks and socio-economic impacts,” in Cities of the 21st Century: New Technologies and Spatial Systems , eds J. Brotchie, M. Batty, P. Hall, and P. Newton (Longman: Cheshire).
Bruinsma, F. (2009). The impact of railway station development on urban dynamics: a review of the Amsterdam south axis project. Built Environ. 35, 107–121. doi: 10.2148/benv.35.1.107
Brunello, L. (2018). High-Speed Rail and Access Transit Networks . Cham: Springer. doi: 10.1007/978-3-319-61415-1
PubMed Abstract | CrossRef Full Text | Google Scholar
Caulfield, B., Rock, S., and Ahern, A. (2014). “Equity and fairness in transport planning: the state of play,” in 93rd Annual Meeting of the Transportation Research Board (Washington, DC: Transportation Research Board).
Cervero, R., and Bernick, M. (1996). High-Speed Rail and Development of California's Central Valley . Berkeley: University of California at Berkeley, Institute of Urban and Regional Development.
Ch2m (2018). Ultra-High-Speed Ground Transportation Study . Washington: Washington Department of Transportation.
Chen, C., and Hall, P. (2011). The impacts of high-speed trains on British economic geography: A study of the UK's InterCity 125/225 and its effects. J. Transport Geography 19, 689–704. doi: 10.1016/j.jtrangeo.2010.08.010
Chen, C., Loukaitou-Sideris, A., De Ureña, J., and Vickerman, R. (2019). Spatial short and long-term implications and planning challenges of high-speed rail: A literature review framework for the special issue. Eur. Plan. Stud. 27, 415–433. doi: 10.1080/09654313.2018.1562658
Chen, X., Tang, F., Huang, Z., and Wang, G. (2007). High-speed maglev noise impacts on residents: A case study in Shanghai. Transport. Res. Part D: Trans. Environ. 12, 437–448. doi: 10.1016/j.trd.2007.05.006
Cheng, Y. (2010). High-speed rail in Taiwan: New experience and issues for future development. Transport Policy 17, 51–63. doi: 10.1016/j.tranpol.2009.10.009
Cohn, S. (2019). Hyperloop and Climate Change . Available online at: https://www.hyperlooppartnership.org/single-post/2019/03/12/Hyperloop-and-Climate-Change (accessed July 15, 2020).
Dorsey, B. (2018). Inside a Hyperloop Pod With First-Class Seats, Mood Lighting . Available online at: https://thepointsguy.com/news/step-inside-a-real-hyperloop-pod-with-first-class-seats-and-mood-lighting/ (accessed October 28, 2020).
Edwards, L. K. (1965). High-speed tube transportation. Sci. Am. 213, 30–40 doi: 10.1038/scientificamerican0865-30
FAZ (2020). Vor Köln und Hamburg: Frankfurter Hauptbahnhof mit den meisten Straftaten . Available online at: https://www.faz.net/aktuell/rhein-main/frankfurt/frankfurter-hauptbahnhof-mit-den-meisten-straftaten-16668149.html (accessed October 2, 2020).
Flyvbjerg, B. (2005). Machiavellian megaprojects. Antipode 37, 18–22. doi: 10.1111/j.0066-4812.2005.00471.x
Flyvbjerg, B. (2012). Why mass media matter to planning research. J. Plan. Educ. Res. 32, 169–181. doi: 10.1177/0739456X12441950
Garmendia, M., De Ureña, J., Ribalaygua, C., Leal, J., and Coronado, J. (2008). Urban residential development in isolated small cities that are partially integrated in metropolitan areas by high speed train. Eur. Urban Regional Stud. 15, 249–264. doi: 10.1177/0969776408090415
Garmendia, M., Ribalaygua, C., and Ureña, J. (2012). High speed rail: implication for cities. Cities 29, 26–31. doi: 10.1016/j.cities.2012.06.005
Hall, P. (2009). Magic carpets and seamless webs: opportunities and constraints for high-speed trains in Europe. Built Environ. 35, 59–69. doi: 10.2148/benv.35.1.59
Janić, M. (2018). Multicriteria evaluation of the high-speed rail, trans rapid maglev and hyperloop systems. Transport. Syst. Technol. 4, 5–31. doi: 10.17816/transsyst2018445-31
Janzen, R. (2017). Trans pod ultra-high-speed tube transportation: dynamics of vehicles and infrastructure. Proc. Eng. 199, 8–17. doi: 10.1016/j.proeng.2017.09.142
Jong, M. (2007). Attractiveness of HST Locations . Master thesis. Urban Planning Universiteit van Amsterdam, Amsterdam.
Kamada, M. (1980). “Achievements and future problems of the shinkansen,” in The Shinkansen High-Speed Rail Network of Japan , eds A. Straszak and R. Touch (Pergamon Press).
Kao, T. C., and Lin, C. K. (2007). “Taiwan high speed rail & its impact to regional development,” in Proceedings of the 4th Asian Civil Engineering Coordinating Council (ACECC) International Conference (Taipei, Taiwan).
Kenworthy, J. (2013). Decoupling urban car use and metropolitan GDP growth. World Transport Policy Pract. 19, 7–21
Kenworthy, J. (2021). “Urban structure and its impact on mobility patterns: reducing automobile dependence through polycentrism,” in The Palgrave Encyclopedia of Urban and Regional Futures , ed R. Brears (Cham: Palgrave Springer Nature). doi: 10.1007/978-3-030-51812-7_167-1
CrossRef Full Text
Kenworthy, J., and Laube, F. (2001), Millennium Cities Database for Sustainable Transport (database prepared for the UITP), International Association of Public Transport (UITP), Brussels.
Mclean, N. (2016). Comparative Analysis of the Hyperloop Against High-Speed Rail for Commuting Between Sydney, Canberra and Melbourne . Honours Thesis, School of Mechanical and Mining Engineering. The University of Queensland.
Möbert (2018). The German Housing Market in 2018 . Available online at: https://www.dbresearch.com/PROD/RPS_EN-PROD/PROD0000000000460528/The_German_housing_market_in_2018.pdf?undefined&realload=EJ0CAaxMWugRNa1yt4fvRJ3R4okEzzRNdoDIUOI97FTiVUQJvjLE32NzNtjYLDaaODjAhfm9Glw1qXjBINO2Q== (accessed November 26, 2020).
Monbiot, G. (2015). Embracing Revolution on Climate Change and Neoliberalism | Letters . Available online at: https://www.theguardian.com/environment/2019/apr/26/embracing-revolution-on-climate-change-and-neoliberalism (accessed February 6, 2020).
Moyano, A., Rivas, A., and Coronado, J. (2019). Business and tourism high-speed rail same-day trips: factors influencing the efficiency of high-speed rail links for Spanish cities. Eur. Plan. Stud. 27, 533–554. doi: 10.1080/09654313.2018.1562657
Muller, P. O. (2004). “Transportation and urban form-stages in the spatial evolution of the American metropolis,” in The Geography of Urban Transportation , eds S. Hanson and G. Giuliano (New York: The Guilford Press).
Murakami, J., and Cervero, R. (2010). California High-Speed Rail and Economic Development: Station-Area Market Profiles and Public Policy Responses . Berkeley: Mimeo, University of California Transportation Centre.
Musk, E. (2013). Hyperloop Alpha . Available online at: https://www.spacex.com/sites/spacex/files/hyperloop_alpha.pdf (accessed February 6, 2020).
Nikitas, A., Kougias, I., Alyavina, E., and Njoya Tchouamou, E. (2017). How can autonomous and connected vehicles, electromobility, BRT, hyperloop, shared use mobility and mobility as a service shape transport futures for the context of smart cities? Urban Sci. 36,40036. doi: 10.3390/urbansci1040036
Oborne, D. (1978). Passenger comfort — an overview. Appl. Ergo. 9, 131–136. doi: 10.1016/0003-6870(78)90002-9
Okabe, S. (1980). “Impact of the sanyo shinkansen on local communities,” in The Shinkansen High-Speed Rail Network of Japan , eds A. Straszak and R. Touch (Belgium: Pergamon Press).
Özbek, R., and Çodur, M. (2021). Comparison of hyperloop and existing transport vehicles in terms of security and costs. Modern Transport. Syst. Technol. 7, 5–29. doi: 10.17816/transsyst2021735-29
Park, D. (2019). Incheon airport maglev line. Przeglad Elektrotechniczny 1, 3–5. doi: 10.15199/48.2019.06.01
Pérez, M. (2019). HYPERLOOP: An Analysis of Its Fit in the European Union . Postgraduate. Politechnico Di Milano.
Peters, D. (2009). The renaissance of inner-city rail station areas: a key element in contemporary urban restructuring dynamics. Crit. Plan. 16, 163–185. Available online at: https://www.researchgate.net/publication/228385956_The_renaissance_of_inner-city_rail_station_areas_a_key_element_in_contemporary_urban_restructuring_dynamics
Pol, P. (2002). A Renaissance of Stations, Railways and Cities: Economic Effects, Development Strategies and Organizational Issues of European High-Speed-Train Stations . Delft University Press, Netherlands.
Preston, J., and Wall, G. (2008). The Ex-ante and Ex-post economic and social impacts of the introduction of high-speed trains in South East England. Plan. Pract. Res. 23, 403–422. doi: 10.1080/02697450802423641
Rajendran, S., and Harper, A. (2020). A simulation-based approach to provide insights on Hyperloop network operations. Transport. Res. Interdisc. Perspect. 4, 1–10. doi: 10.1016/j.trip.2020.100092
Ravenscroft, T. (2019). India Approves Mumbai-Pune Hyperloop In Bid to Build World's First System. Available online at: https://www.dezeen.com/2019/08/07/mumbai-pune-hyperloop-virgin-maharashtra-india/ (accessed September 12, 2020).
Rodrigue, J. (2013). HS2: Mixed Success for High Speed Rail Worldwide . Available online at: https://theconversation.com/hs2-mixed-success-for-high-speed-rail-worldwide-20045 (accessed November 4, 2020).
Roy, A. (1999). The Cost of Living . London: Flamingo.
Roy, A. (2013). The Algebra of Infinite Justice . New Delhi: Penguin Books.
Salet, W. G. M., and Majoor, S. J. H. (2005). “Reshaping Urbanity in the Amsterdam region,” in Amsterdam Zuidas - European Space , eds W. G. M. Salet and S. J. H. Majoor (Uitgevers: Rotterdam).
Sideris, A. L., Cuff, D., Higgins, T., and Linovski, O. (2012). Impact of high-speed rail stations on local development: a delphi survey. Built Environ. 38, 51–70. doi: 10.2148/benv.38.1.51
Taylor, C., Hyde, D., and Barr, L. (2016). Hyperloop Commercial Feasibility Analysis: High Level Overview . Cambridge, CA: Volpe (US Department of Transport).
Trip, J. J. (2004). “The contribution of HST-related development projects to a competitive Urban climate,” in Paper presented to City Futures Conference (Chicago).
Upbin, B. (2016). We Ran the Numbers on a European Hyperloop — And They Look Fantastic . Available online at: https://hyperloop-one.com/blog/we-ran-the-numbers-on-euro-hyperloop (accessed January 5, 2020).
Vale, D. (2015). Transit-oriented development, integration of land use and transport, and pedestrian accessibility: Combining node-place model with pedestrian shed ratio to evaluate and classify station areas in Lisbon. J. Transport Geography 45, 70–80. doi: 10.1016/j.jtrangeo.2015.04.009
van Goeverden, K., Milakis, D., Janic, M., and Konings, R. (2018). Analysis and modelling of performances of the HL (Hyperloop) transport system. Eur. Transport Res. Rev. 10, 1–17. doi: 10.1186/s12544-018-0312-x
Walker, R. (2018). Hyperloop: Cutting Through the Hype . Available online at: https://trl.co.uk/sites/default/files/Hyperloop%20white%20paper_0.pdf (accessed February 5, 2020).
WSDOT (2018). Ultra-High Speed Ground Transportation Study . Available online at: https://www.wsdot.wa.gov/publications/fulltext/LegReports/17-19/UltraHighSpeedGroundTransportation_FINAL.pdf (accessed October 1, 2021).
Yang, J., Guo, A., Li, X., and Huang, T. (2018). Study of the impact of a high-speed railway opening on China's accessibility pattern and spatial equality. Sustainability 10:2943. doi: 10.3390/su10082943
Yin, M., Bertolini, L., and Duan, J. (2015). The effects of the high-speed railway on urban development: International experience and potential implications for China. Prog. Plan. 98, 1–52. doi: 10.1016/j.progress.2013.11.001
Zemp, S., Stauffacher, M., Lang, D., and Scholz, R. (2011). Generic functions of railway stations - a conceptual basis for the development of common system understanding and assessment criteria. Transport Policy 18,7. doi: 10.1016/j.tranpol.2010.09.007
Zhang, H., Li, X., Liu, X., Chen, Y., Ou, J., Niu, N., et al. (2019). Will the development of a high-speed railway have impacts on land use patterns in China? Ann. Am. Assoc. Geograph. 109, 979–1005. doi: 10.1080/24694452.2018.1500438
Zhong, C., Bel, G., and Warner, M. (2014). High-speed rail accessibility: a comparative analysis of urban access in Los Angeles, San Francisco, Madrid, and Barcelona. Eur. J. Transport Infrastructure Res. 14, 468–488. doi: 10.18757/ejtir.2014.14.4.3048
Zidane, Y., Johansen, A., and Ekambaram, A. (2013). Megaprojects-challenges and lessons learned. Proc. Soc. Behav. Sci. 74, 349–357. doi: 10.1016/j.sbspro.2013.03.041
Keywords: hyperloop, high speed ground transportation, economic factors, environmental impact (EI), urban and transport planning, technical factors, ultra-high speed (UHS), human impact
Citation: Premsagar S and Kenworthy J (2022) A Critical Review of Hyperloop (Ultra-High Speed Rail) Technology: Urban and Transport Planning, Technical, Environmental, Economic, and Human Considerations. Front. Sustain. Cities 4:842245. doi: 10.3389/frsc.2022.842245
Received: 23 December 2021; Accepted: 25 February 2022; Published: 04 May 2022.
Reviewed by:
Copyright © 2022 Premsagar and Kenworthy. This is an open-access article distributed under the terms of the Creative Commons Attribution License (CC BY) . The use, distribution or reproduction in other forums is permitted, provided the original author(s) and the copyright owner(s) are credited and that the original publication in this journal is cited, in accordance with accepted academic practice. No use, distribution or reproduction is permitted which does not comply with these terms.
*Correspondence: Jeffrey Kenworthy, j.kenworthy@curtin.edu.au
This article is part of the Research Topic
Renewable Energy Systems for Sustainable Cities
An Open Access Journal
- Original Paper
- Open access
- Published: 03 September 2018
Analysis and modelling of performances of the HL (Hyperloop) transport system
- Kees van Goeverden ORCID: orcid.org/0000-0002-1880-4731 1 ,
- Dimitris Milakis 1 ,
- Milan Janic 1 &
- Rob Konings 2
European Transport Research Review volume 10 , Article number: 41 ( 2018 ) Cite this article
19k Accesses
43 Citations
27 Altmetric
Metrics details
Introduction
Hyperloop (HL) is presented as an efficient alternative of HSR (High Speed Rail) and APT (Air Passenger Transport) systems for long-distance passenger transport. This paper explores the performances of HL and compares these performances to HSR and APT.
The following performances of the HL system are analytically modeled and compared to HSR and APT: (i) operational performance; (ii) financial performance; (iii) social/environmental performance.
The main operational result is that the capacity of HL is low which implies a low utilization of the infrastructure. Because the infrastructure costs dominate the total costs, the costs per passenger km are high compared to those for HSR and APT. The HL performs very well regarding the social/environmental aspects because of low energy use, no GHG emissions and hardly any noise. The safety performance needs further consideration.
Conclusions
The HL system is promising for relieving the environmental pressure of long-distance travelling, but has disadvantages regarding the operational and financial performances.
1 Introduction
The competition between contemporary transport modes has been rather constant over the past decades. However, this has not applied to the European long-distance passenger transport where the airlines have increased their market share substantively. Van Goeverden et al. [ 1 ] have estimated that air travel increased by about 45% between 2001 and 2013 while usage of the alternative modes has been rather stable (car, train) or declining (bus). The increasing dominance of air transport has enlarged the environmental impacts of long-distance transport and this trend is expected to continue in the next decades [ 2 , 3 ]. The aircraft high speed in combination with comparatively low fares particularly those offered by low cost carriers has caused that requirements of travellers have become increasingly demanding, thus leading to a pressure on modes to offer high service quality particularly in terms of the shorter travel times, and low fares. In addition, the environmental impact of transport has gained increasing interest, implying a growing concern with the further dominance of air transport and a demand for more environmental-friendly competitive transport alternatives. This is particularly the case since the current transport modes have been trying to adapt their operational, commercial, environmental, and social performances, though being bounded by their technologies. For these technologies, marginal but not radical improvements have been permanently made. Radical new technologies, which could offer significantly better performances, are still rare and so far have not been able to enter the transport market successfully.
The HL (Hyperloop) system is a new transport technology in conceptual stage that is claimed to provide superior performances to HSR (High Speed Rail) and APT (Air Passenger Transport) system, particularly regarding the travel time, transport costs, energy consumption, and transport safety [ 4 ]. So far, studies on HL have focused on enabling technologies of the system such as the electromagnetic levitation [ 5 ], the dynamics of the HL vehicle and the infrastructure [ 6 , 7 , 8 , 9 ], the implications of the HL for bridge dynamics [ 10 ] and the impact of earthquake forces on the HL vehicle [ 11 ]. Decker et al. [ 12 ] explored the feasibility of the HL system focusing on trades between technical/design aspects and the associated cost. Finally, Janić [ 13 , 14 ] analysed multiple performances (e.g. operational, economic, social, and environmental) of high-speed rail and compared them to competing modes, without including HL in his analysis though.
Existing studies have not yet systematically explored the HL system’s performances as compared to other transport modes. This paper aims at filling in this gap in the literature by exploring the operational, financial, and social/environmental performances of the HL system and comparing them with those of the HSR and APT system. The results of such comparison are intended to underpin the discussion about the overall feasibility of the HL system.
In addition to this introductory section, the paper consists of four other sections. Section 2 provides a brief description of the considered HS (High Speed) transport systems - already fully operational HSR and APT and still on the conceptual stage HL system. Section 3 deals with an analysis and analytical modelling of the above-mentioned performances of the three systems. Section 4 gives a comparison of the HL system’s performances with those of the HSR and APT. For such purpose, the inputs for estimating indicators of performances of the latter two systems (HSR and APT) are extracted from the existing secondary sources (references). The final Section (5) summarizes the main conclusions regarding the prospective advantages and disadvantages of the HL system and provides some perspectives on its market opportunities.
2 The HS (high speed) transport systems
In this section, we present an overview of the deployment and main technical characteristics of the three high speed transport systems considered in this study: HSR, ART and HL.
2.1 The HSR (high speed rail) system
The HSR systems have been developing worldwide (Europe, Far East-Asia, and USA -United States of America-) as an actually innovative system within the railway transport mode, particularly as compared to its conventional passenger counterparts. The system has had different definitions in the particular world’s regions. For example: In Japan, the HSR system is called ‘Shinkansen’ (i.e., ‘new trunk line’) whose trains can run at the speed of at least 200 km/hr. The system’s network has been built with the specific technical standards (i.e., dedicated tracks without the level crossings and the standardized and special loading gauge). In Europe the HSR system has included infrastructure specially built and/or upgraded for the HS (High Speed) travel and considered to be a part of the Trans-European rail transport system/network. Respecting the maximum speed, the HSR lines have been categorized as Category I (for the speeds equal to or greater than 250 km/h), Category II (those specially upgraded for the speeds of about 200 km/h), and Category III (those upgraded with particular features resulting from the topographical relief or the town-planning constraints). In China, according to Order No. 34, 2013 from the country’s Ministry of Railways, the HSR system has been considered to be the new built passenger-dedicated lines with (actual or reserved) speed equal to and/or greater than 250 km/h along these lines and 200 km/h along the mixed (passenger and freight) lines. In the USA, the HSR system has mainly been considered as that providing the frequent express services between the major population centres on the distances from 200 to 600 mi (mile) with a few or no intermediate stops, at the speeds of at least 150 mph (mi/h) on the completely grade-separated, dedicated rights-of way lines (1 mi = 1.609 km) [ 14 ]. Table 1 gives an example of developing the HSR networks round the world.
In addition, Fig. 1 shows the development of the passenger transportation in the European HSR network.
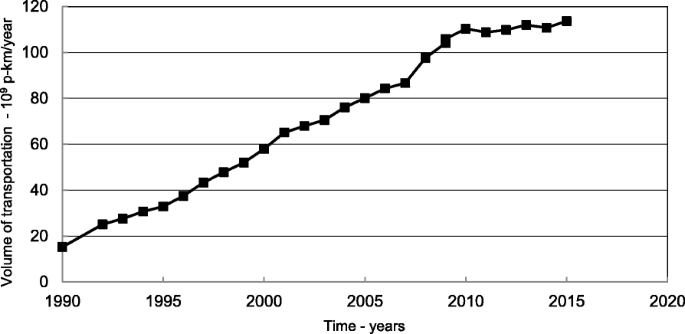
Development of the volumes of passenger transportation in the European HSR network over time (Period: 1990–2015) [ 34 ]
As can be seen, the volumes of transportation in terms of p-km have continuously been growing over the specified period of time, which has been possible thanks to expanding the HSR network in particular European countries.
2.2 The APT (air passenger transport) system
The APT has been permanently growing thanks to improving the ‘aircraft capabilities’, the ‘airline strategy’ and ‘governmental regulation’ (Boeing, 1998). The ‘aircraft capabilities’ has related to increasing speed, payload, and take-off-weight. Both the speed and payload have contributed to an enormous increase in the aircraft productivity, for more than 100 times during the last forty years. In particular, increase in the speed has been noticeable over the last six decades as shown on Fig. 2 .
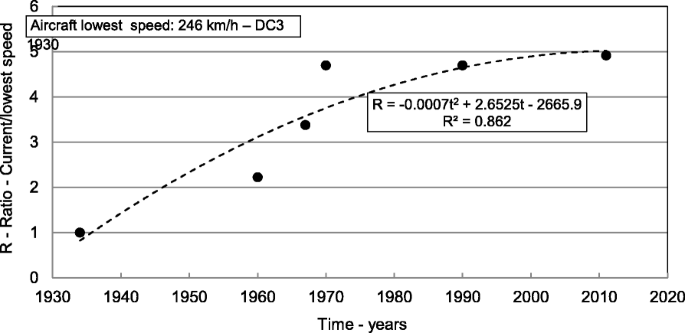
Development of the aircraft speed over time [ 35 ]
During the same period, the aircraft seat capacity has increased from 21 to 32 at the aircraft DC3 to almost 600 at Airbus A380. In addition, the ‘airline strategy’ have permanently deployed bigger, faster, safer, and more fuel-efficient aircraft equipped with lower emission and less-noise engines. As well, the aircraft of various sizes have been progressively engaged to efficiently match markets in the different network configurations, route length, and demand density. The ‘governmental regulation’ has mainly been leading towards liberalization of the national and partially international markets. That in USA (1978) and EU (European Union) (1997) are some of the earliest cases. Consequently, the APT system has been growing over time as shown by the examples on Fig. 3 .
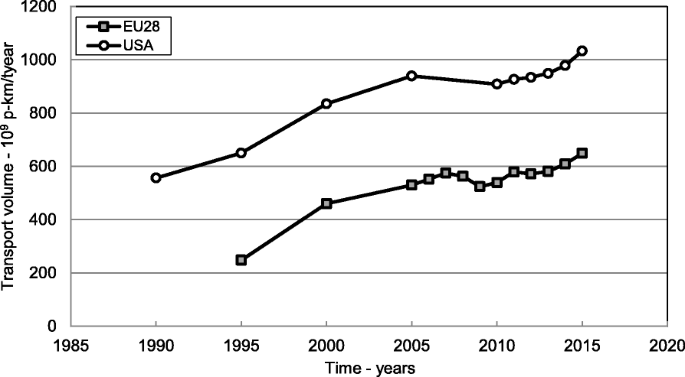
Development of air passenger transport in EU 28 (European Union) and USA (United States of America) over time [ 34 ]
As can be seen, in both areas the volumes of air passenger transportation have been generally growing in the long term, with some fluctuations. As well, the volumes of the world’s air passenger transportation have been growing at an annual rate of 4–6% up to about 7 trillion p-km in the year 2015 [ 15 ].
2.3 The HL (Hyperloop) system
Historically, several pneumatic and maglev trains similar to HL have been proposed at conceptual level primarily aiming to substantially reduce travel time compared to existing modes, and therefore being adopted in the transport system. For example, in 1910 Robert Goddard designed a floating train on magnets inside a vacuumed tunnel that could reach 250 miles/hour covering the distance between Boston and New York in 10 min. In 1972, RAND suggested that a very high speed transit (VHST) system operating in underground evacuated tubes propelled by electromagnetic waves would be technically feasible to travel coast-to-coast in the US in as low as 21 min [ 16 ]. Yet, this report recognized that political feasibility of such project would be very low.
For the purpose of this analysis and modelling its performances, the HL system is assumed to consist of five main components: i) the line/tube including at least two parallel tubes and the stations along them, which enable operations of the HL vehicles in both directions without interfering with each other and embarking and disembarking of passengers, respectively; ii) the fleet of HL vehicles, which can consist of a single and/or few coupled capsules (these are operated by means of a magnetic linear accelerator positioned at the stations, which would accelerate the vehicles/capsules with the support of rotors attached to each of them); iii) the vacuum pumps maintaining the vacuum conditions within the tubes and at the stations at the specified parts; iv) the vehicle control system while operating along the line(s)/tube(s); and v) the maintenance systems for all previous components.
The tubes will be based on elevated pillars except for tunnel sections, while the solar panels above the tubes will provide the system with energy. The ultra-high vacuum approximately at the level of 10 − 8 Torr (British and German standards; Torr = Toricheli) would be maintained in the tube (the atmospheric pressure is variable but standardised at the level of 760 Torr or 1.013·10 5 Pa (Pascal)). Each station of the HL system is to be generally integrated within the tube. It would consist of three modules. The first one is the chamber as a part of the vacuum tube handling the arriving HL vehicle (ultimately ‘arriving’ chamber). After the vehicle enters, de-vacuuming of the chamber is carried out, and the vehicle proceeds to the second module with the normal atmospheric pressure where passengers embark and disembark the vehicle(s). After that, the vehicle(s) passes to the third chamber where at that moment normal atmospheric pressure prevails (ultimately ‘departing’ chamber). Then it spends time until the chamber is de-vacuuming, leaves it, and proceeds along the line/tube. This vehicle handling process takes place at each station of the line. The chambers are separated by the hermetic doors enabling establishing and maintaining the required air pressure in the above-mentioned order.
The capsules would operate in the above-mentioned low-pressure tube(s) on a 0.5–1.3 mm layer of air featuring the pressurized air and the aerodynamic lift as shown on Fig. 4 . Under such conditions, they would be able to reach the maximum speed of v max = 1.220 km/h [ 4 ]; the maximum inertial acceleration would be a + = 0.5 g; g = 9.81 m/s 2 .
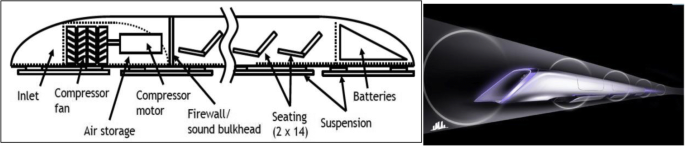
Conceptual design and subsystems of the HL system (source: [ 4 ])
The vacuum pumps are installed to initially evacuate and later maintain the required level of vacuum inside the tubes and in the stations’ first and third chambers. In particular, creating vacuum within the tube implies an initially large-scale evacuation of air and later on removal of the smaller molecules near the tubes’ walls using the heating techniques. These pumps would consume a rather substantive amount of energy. At the initial stage, they would operate until achieving the above-mentioned required level of tube vacuum, then, be automatically stopped, and the vacuum-lock isolation gates opened. In cases of air leakage in some section(s), the corresponding gates will be closed and the pumps activated again. The pumps would be located along the tube(s) in the required number depending on the volumes of air to be evacuated, available time, and their evacuation capacity. As far as de-vacuuming and vacuuming of chambers at the stations is concerned, the required number of vacuum pumps will operate accordingly.
Regarding the characteristics of operations within the tube and at the station(s), the control of safe and efficient movement of vehicles and maintaining the vacuum inside the tube and at the station(s) would be provided by the convenient traffic control and management system.
Given the above-mentioned technical features, the HL is envisioned to be a transport mode for the medium- to long-distance travelling. As such, if operating along the routes without substantive physical barriers, it seems to be a good alternative to APT. At present, the HL technology is being tested in practice on the short test tracks with prototype (capsule) models.
Musk [ 4 ] considers two variants of the HL system: exclusively for passenger only and mixed for both passenger and freight. The latter has larger dimensions for both the tube and the capsules. For example, the diameter of the tube for the exclusive passenger variant is 2.23 m and for the mixed passenger and freight variant is 3.3 m. The capsules for passengers only are of the standardized seating capacity of S = 28 seats/unit, the mixed passenger and freight variant gives room for 14 passengers and 3 full size automobiles per unit. In particular, those intended exclusively to passengers allow them only to sit since the lack of space for walking through the vehicle(s). In addition, the capsules of both variants lack the toilets, which diminishes the flexibility and applicability of the system because the vehicles would need to stop every 30–60 min for a longer time for toilet visits. The capsules of the passenger and freight variant seem to be sufficiently large to enable walking through the vehicle and to visit a toilet when this is built-in. Their frontal area is supposed to be 4.0 m 2 and the height about 1.9 m. The larger dimensions make the system more expensive, but they are essential for its functionality for long-distance travelling. Therefore, our analysis regards the passenger+freight variant with the larger dimensions. Unlike Musk [ 4 ], we assume that it is fully utilized for passenger transport and that the seating capacity is equal to the capacity of the small dimensioned passenger only variant: 28 seats per unit. Room for the toilet can be gained by reducing the luggage compartment. The larger dimensions of the vehicles imply that a larger volume for luggage is available per m 2 area, and that the seating compartment has more room for storing luggage.
3 Modelling performances of the HL, HSR, and APT system
The development and adoption of transport innovations can be influenced by multiple factors. According to [ 17 ] development and adoption of transport innovations is a function of techno-economic, social and political feasibility. If any of those three minimum criteria is not met then the transport innovation will not be adopted. Janic [ 13 ] suggests that the performance of a new transport system can be considered in different ways and from the perspectives of different stakeholders involved, i.e., the users/customers, the transport operator, the governmental authorities at different institutional levels, and the society. If the different interests of stakeholders are not successfully balanced, they may block the implementation of a new transport system. In this study, we explore the operational, financial and social/environmental performances of HL that reflect its all feasibility dimensions (see Fig. 5 ). The performances of its counterparts HSR and APT are considered for the comparative purposes.
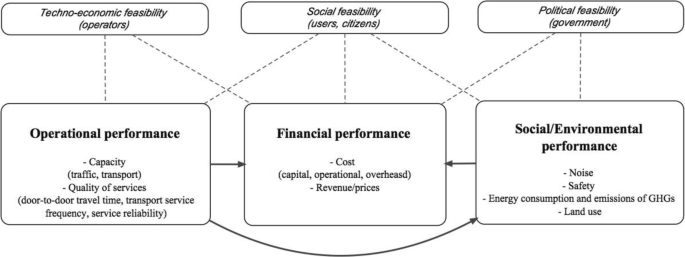
The considered HL, HSR, and APT performances explored in this study
3.1 Operational performance
The operational performance of the HL system generally includes the system capacity and the quality of services. The former is mainly relevant for the operators, and the latter for the users/customers.
3.1.1 Capacity
Similarly to its counterparts, the HSR and APT, the HL system is characterized by its traffic and transport ‘ultimate’ capacity. The ‘ultimate’ capacity is the capacity in the case that everything functions perfectly. In practice, this condition is not met, and the ‘practical’ capacity will be somewhat lower than the ‘ultimate’ capacity.
Traffic capacity
The traffic ‘ultimate’ capacity is defined by the maximum number of vehicles, which can pass through the “reference location” for their counting in one direction during a given period of time under conditions of constant demand for service. In case of the HL system, this is actually the capacity of the infrastructure, i.e., stations, segments between the stations, and the line/tube as the whole.
The ‘ultimate’ capacity of the station (i) of a given HL line/tube can be ‘static’ and ‘dynamic’. The ‘static’ capacity can be defined by the number of tracks/places at the station. The static capacity that is needed to handle the vehicles of guided transport systems during the given period (T) under conditions of constant demand for service can generally be estimated as follows:
is the capacity on the (i-1) segment of the line/tube in terms of the maximum transport service frequency during the time period (T) (veh/min or h); and
is the average time of occupying a track/place at a station (i) by the Hyperloop vehicle (min, h/track).
In the case of the HL system the relation between occupation time and capacity is more complex. The vehicles pass through the three above mentioned chambers, an arriving chamber, a chamber for disembarking and embarking passengers, and a departure chamber. The arriving and departure chambers function as locks. The static capacity is not related to the sum of these occupation times by a vehicle of the three chambers ( τ s/i ), because a) occupation times can overlap (e.g. vehicle 1 can enter the arriving chamber while vehicle 2 is still occupying the platform in chamber 2) –this enlarges the capacity– and b) the arriving and departure chambers are for some time occupied while they are empty (adapting the air pressure for the next vehicle) –this lowers the capacity–. The static capacity of HL can be estimated as:
is the average occupation time of the arriving chamber of station i for one vehicle (min)
is the average occupation time of the platform of a station (i) by one vehicle (min).
is the average occupation time of the departing chamber of station i for one vehicle (min)
Assuming that the occupation times of the two chambers that function as a lock are equal ( τ ca/i = τ cd/i ), the equation can be rewritten as:
is the average occupation time of a lock chamber of station i for one vehicle (min)
The meaning of the eqs. 1 to 3 is, that the number of tracks will be sufficient to handle the vehicles when they run at a frequency that equals the segment capacity. This implies that the station capacity will not be critical for the traffic capacity. However, there may spatial or financial limitations for the number of tracks. One should note that a track includes three chambers and each additional track means building three additional chambers. In that case the station capacity can be critical.
The ‘dynamic’ capacity of the station (i) can be defined by the maximum number of HL vehicles, which can be handled at the given number of tracks/spaces at the station (i) during the given period (T) under conditions of the constant demand for service. Based on Eq. 3 , this can be estimated as follows:
where all symbols are analogous to those in Eq. 3 .
The occupation time of one lock chamber (τ c/i ) in Eq. 4 can be estimated as follows:
is the spatial volume of the first (‘arriving’) and the third (‘departing’) chamber at the station (i) of the line/tube ( i = 1,2,.., N ) (ft 3 or m 3 );
is a binary variable taking the value “1” if a vehicle is in the chamber and the value “0” is the chamber is empty; during the vacuuming and de-vacuuming cycle, both values apply one time;
is the spatial volume of a vehicle (ft 3 or m 3 );
is the initial and final pressure during de-vacuuming and vacuuming the first (‘arriving’) and the third (‘departing’) chamber of the station (i) , respectively (mmHg or Pa (Pascals));
is the number of vacuum pumps at a lock chamber of the station (i) ;
is the capacity of a vacuum pump at the first and the third chamber of the station (i) (ft 3 /min or m 3 /min); and
is the average time of opening and closing the lock gates and disembarking and embarking the HL vehicle in the chamber of the station (i) (min).
Under an assumption that the first and the third chamber at each station are of the same volume, this volume of either of them at the station (i) in Eq. 3 can be estimated as follows:
is the area of the vertical profile of the first (‘arriving’) and the third (‘departing’) chamber (m 2 );
is the maximum number of capsules constituting the HL vehicle per single departure;
is the length of the HL capsule (m);
is the ‘buffer’ distance between the ends of the HL vehicle and the entry and exit door, respectively, of the first and second chamber (m);
is the diameter of the chamber (m).
The volume of the vehicle can be estimated as:
is the frontal area of the capsule (m 2 ).
The occupation time of the platform can be described as;
is the average time that a vehicle stays at the platform for boarding and alighting;
is the minimum time between leaving a vehicle and entering the next at the platform
Line segment(s)
The ‘ultimate’ capacity of the segment (i-1) in front of the station (i) of a given HL line/tube during the period (T) in Eq. 1 can be estimated as follows:
is the minimum time interval between dispatching successive Hyperloop trains along the (i) -th segment of the line/tube in the single direction (min or h);
is the maximum operating speed of a Hyperloop vehicle on the (i) -th segment of the line/tube (km/h); and
is the maximum safe deceleration rate of the Hyperloop vehicle on the (i) -th segment of the line/tube (m/s 2 ).
Equation 9 assumes that for safety reasons in each pair of successive HL vehicle(s) moving in the same direction the leading vehicle needs to be separated by at least the minimum breaking distance of the following vehicle.
The line/tube capacity is the traffic capacity of the HL system and is defined as the lowest of the station and segment capacities. From Eqs. 4 and 9, the ‘ultimate’ capacity of a given HL line/tube in the single direction can be estimated as follows:
where all symbols are analogous to those in the previous Eqs.
Eq. 10 indicates that the ‘ultimate’ capacity of a given HL line/tube is determined by the minimum ‘ultimate’ capacity of its (“critical”) segment(s) and/or the station(s). The ‘ultimate’ capacity is higher than the ‘practical’ capacity. The latter can be described as:
is the practical traffic capacity;
is the utilisation rate of the ultimate traffic capacity
Transport capacity
The transport ‘ultimate’ capacity of a given HL line/tube can be expressed by the maximum number of offered seats in the single direction during the specified period of time (T) . Based on Eq. 10 , it can be estimated as follows:
is the number of seats per capsule (seats/capsule).
The other symbols are analogous to those in Eqs. 6 and 10.
The practical transport capacity can be described as:
is the practical transport capacity;
is the average load factor of the vehicles (the ‘utilisation rate’ of the ultimate vehicle capacity)
For the practical applications, the actual transport service frequency instead of the ‘ultimate’ transport capacity of a HL line/tube in Eq. 10 needs to be considered. This frequency generally depends on the volumes of demand, the HL vehicle’s average seating capacity per departure, and the average preferred load factor as follows:
is the user/passenger demand during the period (T) in single direction (pass/h or pass/day);
The other symbols are analogous to those in Eq. 13 .
The meaning of Eq. 14 is that if the frequency is set equal to the practical traffic capacity ( μ(T)* ), the transport capacity can be superfluous compared to the demand. That could be a reason to provide services with a lower frequency. In that case, the (scheduled) service frequency can in some cases depend on a policy regarding a ‘decency’ transport service frequency.
Technical productivity
Multiplied by the average vehicle operating speed along the line/tube the transport capacity gives an estimate of the technical productivity of a HL system under given conditions. From Eqs. 10 and 14 , this maximum technical productivity is equal to:
is the average speed of the HL vehicle(s) along the line/tube in the single direction (km/h).
The other symbols are analogous to those in the previous Eqs.
One can conclude from Eqs. 11 and 14 that f(T,Q) never can exceed μ(T) . Eq. 15 can then be rewritten as:
The average speed ( \( \overline{v} \) ) of the HL vehicle(s) in Eq. 16 can be estimated as follows:
is the length of a given HL line/tube (km); and
is the average turnaround time of the vehicles/capsules (min)
The other symbols are analogous to those in previous Eqs. ( \( L=\sum \limits_{i=1}^{N-1}{l}_i \) ) .
The technical productivity in Eq. 16 can also be estimated analogously.
Based on Eq. 3 , the total time, which the HL vehicle(s) would spend at all stations along the line while moving in the same direction, is estimated as:
is the number of stations along the line/tube including the begin and end station (terminuses); and
is the average time, which the HL vehicle spends at the begin and the end station (terminus), respectively (min/veh).
τ s/i is the passing time of a vehicle through the station (see also Eq. 1 ), This time is equal to:
The other symbols are analogous to those in Eq. 3 .
The running time of the HL vehicle(s) along the line/tube in the single direction is estimated as follows:
is the maximum operating speed of the vehicle along the (i) - the segment of the line (km/h); and
is the maximum safe deceleration and acceleration rate, respectively, of the HL vehicle(s) on the (i) - the segment of the line (m/s 2 ).
The total turnaround time of the HL vehicle along the line can be estimated based on Eqs. 18 and 20 as follows:
Given the transport ‘ultimate’ capacity of a given line/tube (μ(T)) in Eq. 10 or the transport service frequency in Eq. 14 , and the average turnaround time per vehicle (τ) in Eq. 21 , the required size of the HL fleet (Total number of capsules) can be estimated as follows:
3.1.2 Quality of services
The quality of services influences (in addition to fares) the attractiveness of the HL system services and as such indicates its relative advantage/disadvantage over the competing modes such as HSR and APT. The relative advantage can be seen as the degree to which an innovation is perceived better than the product it replaces or competes with [ 18 ]. The relative advantage has considered to be one of the strongest predictors of the outcome of the decision on whether or not to adopt the innovation. In general, a new transport system does not need to perform better on all aspects, but overall - taking all the relevant characteristics of the service into account - it should offer some added value, i.e., benefits to its users/passengers. In the given context, the attributes of quality service of the HL system such as a) door-to-door travel time; b) transport service frequency; and c) reliability of services are considered relevant for eventual mode/system choice.
Door-to-door travel time
The door-to-door travel time consists of the access and egress time, schedule delay (including possible time for luggage checking) at the boarding and alighting stations, in-vehicle time, and the interchange time between different HL vehicles and their particular services at intermediate and end stations.
The access and egress time
The access and egress time depends on the interconnectivity between the HL system and the pre- and post-haulage systems, the density of the HL stations, and the speed of the pre- and post-haulage systems (from the users’ doors to the HL station, and vice versa). The access and egress time generally varies at particular HL stations depending on the local spatial and traffic conditions.
The waiting time
The waiting time depends on the frequency of accessible HL services. If there is no limitation on the accessibility, the waiting time is determined by the schedule delay. Based on Eq. 14 , the schedule delay can be estimated as follows:
where all symbols are as in the previous Eqs.
In the case of full accessibility and frequent and punctual services, the waiting time will be equal to the schedule delay. If the frequency is lower than 6/h, the average waiting time at the station will tend to be smaller than the schedule delay [ 19 ], but then there will be some ‘hidden’ waiting time at the departure location. If seat reservation is obligatory, which is common for long-distance modes, the passengers can use only the service for which they reserved a seat; in that case, the frequency of accessible services is just 1. Particularly in the case of low frequencies, the timetables of connecting scheduled systems as well as risk aversion of travellers for missing the intended service can affect the waiting time.
Low punctuality increases waiting time. The punctuality of the HL system correlates with the homogeneity of successive services (regarding to destination/routing, intermediate stops) and the scheduled buffer times. In the case of a network where some passengers also make interchanges, the policy on whether/how long to wait for the delayed connecting services can additionally affect the punctuality and waiting time.
In-vehicle time and interchange time
The in-vehicle time of the HL system depends on the travel distance, the average speed, and the stopping time at the particular stations.
If the HL system is set up as the network where some travellers also make interchanges within it, the interchange time will depend on the frequency of services, matching the timetables, punctuality, and the policy on waiting for delayed connecting services.
The in-vehicle time and the interchange time correlate with the door-to-door distance. The access/egress and waiting times are ‘fixed ‘times to this respect. The relative values of the latter two time components will decrease when the travel distances increase.
Interchanges
The need to make interchanges generally diminishes the overall quality of service because these may extend travel times and make trips less convenient. In the long-distance travel markets, which the HL system is supposed to penetrate, the users/passengers usually have luggage with them. They generally will have to make at least two interchanges (between the access mode and the HL, and between the HL and the egress mode). In some cases they have to make interchanges within the HL system. The opportunity of interchanges in the access and egress trips is related to the density of HL stations. The opportunity of interchanges within the HL system is related to the design of the HL network.
Transport service frequency
The relevance of the service frequency as perceived by the traveller will depend on the envisaged business plan of HL: either as a ‘walk up’ service (i.e. direct access without reservation in advance) or through an advanced obligatory seat reservation. In a scenario with the advanced seat reservation, on the one hand a lower frequency (i.e. 3–4 dep/h) would be well acceptable, while on the other hand the offered service frequency at the time of booking will be lower than the scheduled frequency in the case services are fully booked. In case of ‘walk up’ services the service frequency can also be lower than the scheduled frequency, i.e. when the demand exceeds temporally the offered capacity; then the imbalance between demand and supply will increase waiting times [ 20 ].
Service reliability
The HL system has two major characteristics that enable a potential high reliability of its services. This is a completely automated system, which as such, per definition, excludes delays due to the human errors. In addition, HL system operates in a closed environment which makes it resilient to the weather conditions. Of course, like any other transport system, the reliability of the HL transport services will depend on the technical reliability of all parts of the system (i.e., capsules, infrastructure, and control system).
Table 2 gives the very preliminary estimates of the above-mentioned indicators of operational performances for three considered systems - HL, HSR, and APT using the above-mentioned analytical models. Mode specific assumptions are presented below the table in the form of notes.
As can be seen, based on the technical characteristics of the HL, its transport service frequency is estimated to be 12 dep/h, which is comparable to that of HSR. In addition, under given conditions, the HL system would perform better than its HSR and APT system counterpart only in terms of the indicator - the total station-station travel time.
The station capacity depends on the choices of pumping capacity and number of tracks. Based on Eq. 5 , the pumping capacity for one lock chamber that makes the capacity of the chamber equal to the segment capacity can be calculated. Assuming one track, the required capacity can be described as:
Assuming that the chamber diameter is equal to the tube diameter (Δ = 3.3 m), and that m = 1, d = 30 m, B = 3 m, k c = 0.5 (average of 0 and 1), A c = 4.0 m 2 , P 1 i = 0.74·1.013·10 5 Pa (Equivalent to the altitude of 2500 m MSL (Middle-Sea-Level)), P 2i = 1·10– 10 Pa (Ultra High Vacuum), f = 12/h, U i = 0.8 and τ 0i = 1 min, the required pumping capacity is about 5000 m 3 /min, e.g. 10 pumps ( n i = 10) that produce 500 m 3 /min each ( C i = 500). If more tracks are built, the calculated pumping capacity should be divided by the number of tracks.
3.2 Financial performance
Similarly as at the other transport modes and their systems, the financial performance of the HL system is defined by its revenues, costs, and profits as the difference between the former two. Consequently, the zero profitability achieved by the competitive prices given the costs could guarantee the bottom line for a stable economic viability of the HL system.
3.2.1 Costs
The costs consist of capital costs, operational costs, and overhead costs. The capital costs are the costs for building the infrastructure (tracks, stations), and the costs for purchasing the vehicles. The operational costs regard the cost of maintenance of infrastructure and vehicles, and the costs related to the operation of the vehicles and stations. The overhead costs comprise the capital and maintenance cost of real estate, and the staff costs.
The estimation of the costs of a still not existing system is a rather complex task. Therefore, in the given context, these costs are estimated based on published figures regarding the actual costs of the Maglev-system that are – to a certain extent – comparable to that of the HL system [ 21 ].
The cost level is defined by the cost value, currency, and time. One Euro in 2010 reflects a different cost level than either one US Dollar in 2010 or one Euro in 2015. For the sake of comparability, we will convert the figures to Euros of 2015.
Capital cost for building tracks
The capital cost for building 1 km of line/tube is likely to depend largely on the local conditions. Building in an empty area on flat sandy soil will be cheaper than building in a highly urbanized area, in moorland, or in mountains. Crossing wide rivers or the need to build tunnels will increase the costs. Musk [ 4 ] has estimated the costs of tubes on pylons and tubes in tunnels amounted €10.3 million/km and €34.0 million/km, respectively, for the passenger + freight variant (converted into 2015€).
For the purpose of comparison, there is the example of a high-speed Maglev connection between Shanghai Pudong airport and the outskirts of the city in the form of a dual track of the length of 30 km and two stations (begin and end). Published costs are $1.2 billion and $1.33 billion [ 22 , 23 ] (2002$US). A possible explanation for the difference is the exclusion/inclusion of the two stations. Both amounts included the purchase cost of the vehicles. Excluding station costs and vehicle costs, the investment costs would have been about €41 million per km track (€2015). Cost estimates for an extension of the line to Shanghai Hongqiao Airport were just the half: about €20 million €/km [ 24 ]. A reported reason for the lower costs has been using all-concrete modular design that would reduce the cost by 30%. A second possible reason for the lower cost has been a more solid soil. The current track has been built in an area with seismic activity and weak alluvial soil. This has required the construction on piles, which raised the costs. Another cost estimate of 34 million AU$/km (2008) or 26 million €/km (2015) relates to the proposed Maglev line in the Melbourne area [ 25 ]. This estimate is somewhat higher than that for the Shanghai extension. Considering that the cost estimates generally are too low and therefore the Melbourne estimate might be more realistic than the Shanghai estimate, it is assumed that the costs of the Maglev track are in the order of 25 million €/km under favourable conditions.
The costs of 1 km of the HL line/tube will likely be somewhat higher than the cost of Maglev because the latter system does not have the costs for tube construction and the costs for vacuum pumps. On the other hand, the HL does not need the concrete guideway unlike the Maglev. Consequently, it is assumed that the construction costs of the two systems are similar and therefore adopted to be 25 million €/km for the HL system built on solid soil This appears more than double the costs that were estimated by Musk [ 4 ] .
Assuming that the actual cost of 40 million €/km for the current Maglev track built on weak soil could have been reduced to about 35 million €/km by using a modular design, the latter figure is adopted for the HL system as well.
The estimated costs for building tunnels at the HL system of 34.0 million €/km [ 4 ] can only be compared with the corresponding costs of the railway or road tunnels – the Gotthard base tunnel consisting of two single-track tunnels: 200 million €/km [ 26 ]; the Chuo Shinkansen railway line in Japan between Tokyo and Nagoya where 60% of the line goes through tunnels: 160 million €/km [ 27 ]; the Channel tunnel between France and Britain of the length of 50.5 km: 4.65 billion £/km (1990) or 190 million €/km (2015) [ 28 ]. These figures indicate that the tunnel costs for a double track railway line are in the order of 200 million €/km. This is likely considerably higher than the corresponding costs at the HL system. One of the main reasons is a much smaller diameter of the HL tube – for example, the two single-track Gotthard tunnels with diameters of about 9 m vs a HL tube of 3.3 m. The tunnel construction costs for two HL tubes might then even be somewhat lower than the costs for one single-track rail tunnel. If it is assumed that the costs were underestimated by about a factor 2, just like the argued underestimation for the tube on pylons, the real costs for two parallel tubes would be in the order of 70 million €/km.
Capital cost for building stations/terminals
The building costs for a station/terminal were estimated to be about 125 million $US (116 million €). The costs for the two stations of the current Maglev line near Shanghai could be 130 million US$ for two stations (i.e., 77 million €/station), which is significantly lower than the above-mentioned amount [ 4 ]. However, the HL system’s stations are more complex than that of the Maglev system because they should give access to vehicles in the evacuated tubes as mentioned above. Therefore, it is assumed that the cost per station of the HL system of €116 million is a fairly good estimate. Stations at nodes of the network where several lines inter-connect will likely be more expensive.
Costs of vehicles
The costs for purchase of a vehicle (capsule) were estimated to be about €1.42 million [ 4 ]. These are the costs of a vehicle without toilets. Adding a toilet is supposed to increase the costs to about €1.52 million. For the purpose of comparison, the cost of one carriage of a Maglev train with the capacity of 90 seats are €12.5–15 million (compared to the capacity of the HL capsule of 28 seats) [ 25 ]. The average unit cost per seat which might be rather comparable are €0.14–0.17 million for the Maglev and €0.054 million estimated for the HL. In the present case, it is assumed that the average unit cost for the HL capsule is 0.17 million €/seat, which is more than the threefold of the above-mentioned estimation by Musk [ 4 ]. The assumed cost of a capsule is then €4.8 million.
The annual costs
The capital costs discussed above as incidental costs can be calculated as the annual costs (depreciation and interest) as follows:
is the annual capital cost of the cost element e (€/track, station, and/or vehicle);
is the incidental capital cost of the cost element e (€);
is the residual value of cost element e (€);
is the life span of infrastructure element e (years); and
is the interest rate (%/year).
Table 3 gives an overview of the incidental investment and the annual costs for the HL system. In all cases, it is assumed no residual value ( R e = 0) for all cost elements, the interest rate: I t = 4%/year, and the life spans as an average used in the EU-countries for the rail and road infrastructure and rolling stock [ 29 ].
Maintenance costs of infrastructure and rolling stock
For the maintenance costs of the HL lines/tubes, stations, and rolling stock, a fixed ratio to the capital costs is assumed. The World Bank [ 30 ] states that the variable component of rail infrastructure cost can vary from just a few percent to about 30% depending on the intensity of use. The HL system is assumed to be heavily used, leading to relatively high maintenance cost, but the ratio to the capital cost will be smaller than for rail because of the lack of physical contact between the vehicles and the infrastructure. Consequently, the ratio of 10% is assumed for both infrastructure and vehicles setting the annual maintenance costs at 10% of the annual capital costs.
Operating costs
The operating costs consist of the costs for staff in the vehicles and at the stations, and the traffic management costs. Generally the energy costs for moving the vehicles are also part of the operating costs, but the HL is a special case because it is assumed to take energy from the solar panels at the top of the tube. Some estimates indicate that such produced energy exceeds the energy consumption by the vehicles [ 4 ]. The capital and maintenance costs of the solar panels and the transmission of energy to the vehicles are then the only energy costs.
The costs for employees in the vehicles and stations depend on the organization, i.e., the number of employees in the vehicles, and manpower needed for ticket sales and control. In the present context, it is assumed that in each capsule one employee is present checking the seat belts, helping in the case of problems, and possibly providing some food and drink. The staff at stations would include two employees per station controlling and possibly selling tickets, and helping and guiding passengers. Assuming that the average operation time of a capsule is 15 h/day, that stations are opened for 18 h/day, and that the average working time of an employee is 7 h/day (including holiday and sickness absence), the number of full-time employees for a single capsule is 2.14 and for a station 5.14. Assuming an average annual wage of €35,000, the annual operation cost for one capsule would be €75,000 and for a station €180,000. These costs appear to be relatively small compared to the capital cost.
The traffic management costs depend on the intensity of use and the complexity of the network. It is assumed that these costs are equal to the wage of one employee for each 1000 km of ‘double tube’. Assuming an operation time of 18 h per day, 2,57 full employees are needed per 1000 km of the line/tube. The relating annual costs would be €90,000/1000 km, or €90/km.
Overhead costs
The overhead costs include the capital and maintenance cost of real estate, and the staff costs. In the present context, it is assumed that the real estate costs are marginal compared to the capital and maintenance costs of the HL infrastructure. As such they are neglected. As far as the staff costs, one overhead employee is assumed per each ten employees needed for operation, these costs are included by increasing the costs of operational staff for 10%.
Overview of the costs
Table 4 gives an overview of the annual unit costs of the HL system. At vehicles, the costs are also expressed per seat and seat-km, which makes them comparable to that of other transport systems. For the calculation of numbers per seat km, we assume 28 seats per capsule, 15 operating hours per day per capsule, and an average distance of 600 km per hour in the operating period.
The vehicle cost per seat-km is very low compared to the vehicle costs of other systems. Earlier calculations indicated that these costs ranged from 0.022–0,058 €/s-km (1993€) at different public transport systems in the Netherlands [ 31 ]. These costs would be even higher when expressed in 2015€, but public transport provision has become more cost-efficient since. An interesting finding in the study was that the costs are negatively correlated to the speed of a system. The explanation is the fact that most cost components are time related, like the salary of the staff, which lowers the cost per km when the speed increases. Very low costs for the extremely fast HL system could then be expected. An additional explanation is that the energy costs – the only cost component where the per-km cost increases with distance– are not included in the HL vehicle costs.
3.2.2 Revenues/prices
At an economically viable transport system as the HL system intends to be, the average unit price that the users/passengers pay should at least cover the corresponding total average unit cost. These costs depend on the local conditions and the configuration of the system, including
Soil condition, natural barriers; the impact is illustrated in Table 4 .
Average station spacing.
Connectivity; this defines together with the station spacing the number of stations per km track that has to be built; in the case of just one line connecting two stations, the number of stations per km at a given station spacing is about two times the number in a large network.
Frequency of the services; when the service frequency increases, the infrastructure costs are divided among more services and will be lower per ride.
Load factor of the vehicles; this is inversely linearly correlated with the costs per passenger; because of the low transport capacity of the HL (see Table 2 ) and the high market potential because of the very high speed (even higher than the airplane), generally a high load factor might be expected [ 32 ].
The costs for the track infra make up the major part of the costs. This implies a strong relation between service frequency and costs per person km. Figure 6 shows this relation for three types of local conditions, starting from the costs in Table 4 . Assumptions are a station spacing of 500 km, a high network connectivity, and a load factor of 80%. The indicated frequencies range from 15 to 216 dep/day/direction, which is the maximum capacity based on the traffic capacity of μ = 12 dep/h (Table 2 ) under conditions of operation during 18 h/day.
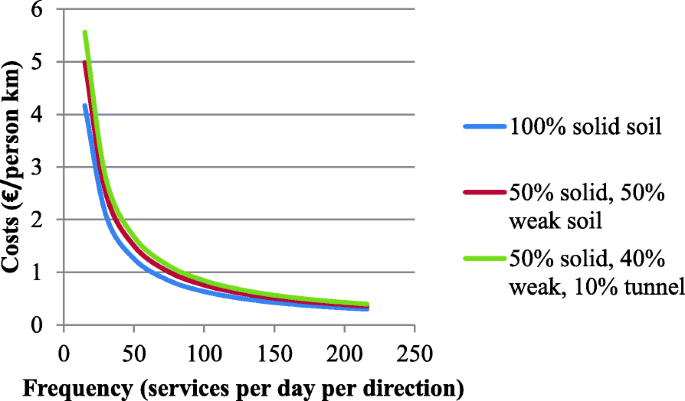
Relation between the average unit costs and transport service frequency
The lowest costs (and the cost-meeting fares) in the figure, in the case of 216 services per day, are €0,30 in the case of solid soil along the whole route, €0,36 in the case of 50% solid soil and 50% weak soil, and €0,40 in the case of 50% solid soil, 40% weak soil, and 10% tunnel. A comparison with the fares of alternative modes is difficult because there is generally a wide range of fares for the same route because of revenue management. Based on the experiences of De Decker [ 33 ], the most common fares for high-speed trains would be between €0,15 and €0,25 per km, and those for conventional long-distance trains about half the HSR fares. The fares for low-cost airlines are also significantly lower than the HSR fares.
We remind the reader that the HL cost calculations regard the system with larger dimensions that enables to walk through the vehicles and visit the toilet. These costs could be 20–30% higher than those for a smaller system where passengers only can sit (according to Musk [ 4 ] who calculated figures for the two systems). A small-dimensioned system would still be expensive compared to the other long-distance travel modes.
Cost calculations for a still not existing transport system are inevitably highly uncertain. However, the cost calculations produce one strong result: the costs are predominantly determined by the costs for the track infra, and therefore a high service frequency combined with a high load factor is needed for cost-meeting fares that are more or less competitive to those of the alternative transport modes.
3.3 Social/environmental performance
The indicators of the social/environmental performances of the HL system include noise, safety, energy consumption and related emissions of GHGs (Green House Gases), and land use.
3.3.1 Noise
Noise produced by transport vehicles can cause annoyance and harmful effects to people living and/or working close to transport routes. Moreover, traffic noise limits the possible use of space along the routes, and hence may cause an opportunity cost regarding land use. The impact of noise depends on the noise levels at sources, the number of people exposed to them, and duration of the noise exposure. This implies that this performance at the considered systems mainly depends on the routing of transport lines and speed and number of passing by vehicles.
The HL is supposed to hardly produce any external noise affecting relatively close population. This is due to the fact that the HL is not in contact with the tube and therefore there is no transfer of vibration. Any noise from the capsule itself will not be heard outside the tube and the low air pressure inside the tube prevents noise from moving the capsule. The only potential source of noise could be the vacuum pumps, but these are assumed to produce negligible noise [ 21 ].
3.3.2 Safety
In evaluating the performance of a transport system a distinction is usually made regarding the internal and external safety. The internal safety relates to risk and damage caused by incidents to the users/operator of the transport system itself. The external safety reflects the possible risk and damage of accidents/incidents to people and their living/working environment outside the systems. The HL system is a dedicated and closed transport system, excluding any kind of interaction with other transport modes and its direct environment. Hence there are no external safety concerns, giving the HL system seemingly an advantage over its prospective counterparts - APT and HSR.
Internal safety benefits are expected because the HL system is a completely automated system and hence excludes the possibility of human errors. In addition the HL system is supposed to be designed according to the fail-safe-principle: in case of danger (e.g., a rapid depressurization in the capsule or tunnel), the “clever” systems will stop the capsule and, if needed, will provide means of individual salvation (e.g., oxygen masks for passengers). However, many safety issues still need further consideration, elaboration and testing, such as for example, evacuation of people, stranded capsules, incorporation of emergency exits, etc. [ 20 ].
3.3.3 Energy consumption and emissions of GHGs (green house gases)
The HL system is expected to be less energy demanding compared to the HSR mainly due to having less friction with the track(s) and low air resistance due to the low pressure in the tube. Some preliminary estimates have suggested that the HL system can be about 2–3 times more energy-efficient than the HSR, and depending on transport distances, about 3–6 times more energy-efficient than APT [ 20 ]. This is mainly because the HL system is intended to be completely propelled by the electrical energy obtained by the solar panels on top of the tube(s). These are claimed to be able to generate more than the energy needed to operate the system. This also takes into account that sufficient energy can be stored (e.g., in the battery packs on board the vehicles) to operate the system at night, in periods of cloudy weather, and in tunnels [ 4 ].
In general, emissions of GHGs are directly related to the energy consumption. If only emissions of GHGs by operations are considered, regarding the above-mentioned primary energy source, the HS system will not make any of them. However, the indirect emissions from building the infrastructure (lines and stations/terminals), rolling stock (capsules), and other equipment should be taken into account in cases of dealing with the system’s life-cycle emissions of GHGs.
3.3.4 Land use
In general, land used to facilitate transport systems cannot, except for underground transportation, be used for other purposes, hence creating an opportunity cost. The valuation of land occupied by the HL system will be a function of the space that is needed (width and length of the infrastructure) and the value of the land. The latter will depend heavily on the specific routing of the line. On the one hand, the HL system is planned to be elevated on pillars, so the effective land occupation on the ground (net area of land needed) can be limited. On the other hand, it remains to be seen if the space between the pillars can be used meaningfully. Moreover, the elevated construction may bring along visual pollution. In general the total amount of land (gross area of land) required for new transport infrastructure can be minimized by maintaining the route as close as possible to the existing transport infrastructure. The HL system’s tubes will be mounted side by side on elevated pillars. For the small tubes – designed for passenger transport only – the size of the pillar that carries two tubes is about 3.5 m wide [ 21 ]. The tubes for mixed traffic (passengers and freight) are larger and hence the pillars for these tubes are also expected to be larger, i.e. 5.2 m. Since the pillars will be spaced averagely 30 m and the possibilities to use the space on the ground in between effectively is limited, the net area needed for 1 km of HL system’s line will be about 0.5 ha. The average gross area of taken land by the line is estimated to be about 1.0 ha/km. Despite of more efficient land use of the HL system, it is likely that it will have higher cost of land than, for instance, its HSR counterpart. This is because the HL system is less flexible than HSR system in routing the line particularly in terms of accommodating to the sharp turns.
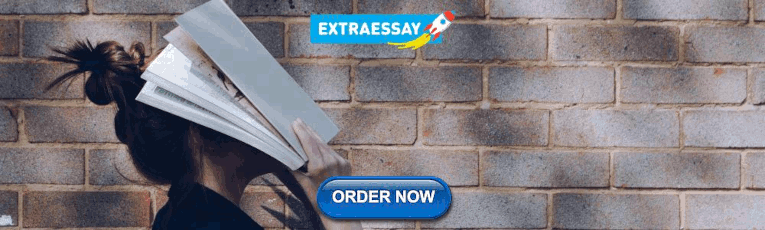
4 Overview of the indicators of performance
An overview of the estimated indicators of performances of the HL, HSR, and APT systems according to the methodology presented in Sections 3.1 to 3.3 is summarized in Table 5 . For those estimations HL, HSR, and APT systems are considered as potential competitors in the medium-distance passenger transport markets(s) of the length of 600 km. Mode-specific assumptions are presented below Table 5 in the form of notes.
As can be seen, some indicators are expressed in quantitative and some others in qualitative terms, the latter based on a relative performance, i.e., relative ranking the transport systems at three levels - low, moderate, and high.
Table 5 demonstrates that the most striking differences between the HL mode and the two other modes regard the vehicle capacity, the vehicle costs per seat km, and the GHG emissions. In all cases, the values are low for HL. The low vehicle capacity results from the assumed short vehicle length. Coupling vehicles would increase the capacity, but moving coupled vehicles through curves might be a technical challenge. Moreover, the chamber lengths have to be increased. The low vehicle costs per seat km can be explained by the extremely high speed (most vehicle costs are correlated with time and not with distance) and the absence of energy costs: the costs for the solar cells are part of the line infrastructure costs. The GHG emissions are low (even zero) because it is assumed that the solar cells provide all energy.
5 Conclusions
Hyperloop (HL) is a new mode of transport that claims to be a competitive and sustainable alternative to the long-distance rail transport (HSR (High Speed Rail)) and the medium-distance APT (Air Passenger Transport) system (less than or equal to 1.500 km). Taking into account that the performance of the HL system can be considered in different ways and from the perspectives of different stakeholders (i.e., passengers, transport operators, government authorities, and society) the operational, financial, social/environmental performances of the HL system have been investigated and evaluated.
In comparing the HL with the HSR and APT system, it has been found that the HL system has relatively positive social/environmental performances, particularly in terms of the energy consumption, emissions of GHGs, and noise. The HL system can potentially be a very safe mode, but both HSR and APT have also a very good safety track record.
A major weak point of the HL system technology appears to be its rather low transport capacity, mainly due to the low seating capacity of individual vehicles/capsules, which affects both the operational and the financial performance. Consequently, the investment costs of HL infrastructure make up a large part of the total costs per seat-kilometre, raising the latter to a higher level than those of its counterparts – HSR and APT. Hence, the break-even fares would also be higher, even if the load factor is relatively high. This finding suggests that HL-application may be limited to the premium passenger transport market, in which there is ‘willingness to pay’ for the strongest feature of HL system service carried out at the very high average speed.
So far, the HL technology is in its infancy and there are still many uncertainties around the system that need further exploration. From operational perspective, an important research issue is if and how the HL system transport capacity could be increased, for instance, by increasing the number seats or coupling several capsules into a single vehicle (‘train’). And also to what extent such change in capacity could influence other operational, financial and socio/environmental performances of the system. An initial study explored the relationship of HL vehicle capacity to total energy consumption and found the former being rather insensitive to the latter (Decker et al., 2017). From financial perspective, further research is needed to more accurately estimate costs associated with HL development especially with respect to infrastructure (i.e. tracks, stations and vehicles) which form the larger part of the total costs per seat-kilometre. Apparently, further specification of these costs requires more research on technological aspects of the system. Finally, from social/environmental perspective, further research is required in exploring the total life-cycle energy consumption and GHGs emissions of the system including infrastructure development (lines and stations/terminals), rolling stock (capsules), and operation of sub-systems such as the vacuum pumps. Moreover, estimation of social performance of the system would be improved by further research on possible implications of HL for social welfare such as accessibility to life-enhancing opportunities and creation of jobs (direct and indirect).
Van Goeverden CD, Van Arem B, Van Nes R (2016) Volume and GHG emissions of long-distance travelling by western Europeans. Transp Res D 45:28–47. https://doi.org/10.1016/j.trd.2015.08.009
Article Google Scholar
Lee DS, Fahey DW, Forster PM, Newton PJ, Wit RCN, Lim LL, Owen B, Sausen R (2009) Aviation and global climate change in the 21st century. Atmos Environ 43:3520–3537. https://doi.org/10.1016/j.atmosenv.2009.04.024
European Commission (2014) EU Energy, Transport and GHG Emissions, Trends to 2050, Reference Scenario 2013. Publications Office of the European Union, Luxembourg
Google Scholar
Musk E (2013) Hyperloop Alpha. SpaceX, Texas http://www.spacex.com/sites/spacex/files/hyperloop_alpha-20130812.pdf
Abdelrahman AS, Sayeed J, Youssef MZ (2018) Hyperloop transportation system: analysis, design, control, and implementation. IEEE Trans Ind Electron 65(9):7427–7436. https://doi.org/10.1109/TIE.2017.2777412
Braun, J, Sousa, J, Pekardan, C (2017) Aerodynamic design and analysis of the hyperloop. AIAA Journal 55(12):4053-60 https://doi.org/10.2514/1.J055634
Chin, JC, Gray, JS, Jones, SM, Berton, JJ (2015) Open-source conceptual sizing models for the hyperloop passenger pod. 56th AIAA/ASCE/AHS/ASC Structures, Structural dynamics, and materials Conference, Kissimmee, Florida. https://doi.org/10.2514/6.2015-1587
Janzen R (2017) TransPod ultra-high-speed tube transportation: dynamics of vehicles and infrastructure. Procedia Engineering 199:8–17 https://doi.org/10.1016/j.proeng.2017.09.142
Yang Y, Wang H, Benedict M, Coleman D (2017) Aerodynamic Simulation of High-Spee Capsule in the Hyperloop System. In: 35th AIAA Applied Aerodynamics Conference. American Institute of Aeronautics and Astronautics, Reston
Alexander NA, Kashani MM (2018) Exploring bridge dynamics for ultra-high-speed, Hyperloop, trains. Structures 14:69–74 https://doi.org/10.1016/j.istruc.2018.02.006
Heaton TH (2017) Inertial forces from earthquakes on a Hyperloop pod. Bull Seismol Soc Am 107(5):2521–2524 https://doi.org/10.1785/0120170054
Decker K, Chin J, Peng A, Summers C, Nguyen G, Oberlander A, Sakib G, Sharifrazi N, Heath C, Gray JS, Falck RD (2017) Conceptual Sizing and Feasibility Study for a Magnetic Plane Concept. In: 55th AIAA Aerospace Sciences Meeting. American Institute of Aeronautics and Astronautics, Reston
Book Google Scholar
Janić M (2003) Multicriteria evaluation of high-speed rail, Transrapid maglev and air passenger transport in Europe. Transp Plan Technol 26(6):491–512 https://doi.org/10.1080/0308106032000167373
Janić M (2016) A multidimensional examination of performances of HSR (high-speed rail) systems. Journal of Modern Transportation 24(1):1–21 https://doi.org/10.1007/s40534-015-0094-y
AIRBUS (2017) Growing Horizons 2017/2036: Global Market Forecast. AIRBUS, S.A.S, Blagnac Cedex, France
Salter RM (1972) The Very High Speed Transit System. RAND Corporation, Santa Monica
Feitelson E, Salomon I (2004) The political economy of transport innovations. In: Beuthe M, Himanen V, Reggiani A, Zamparini L (eds) Transport development and innovations in an evolving world. Springer, Berlin, pp 11–26
Chapter Google Scholar
Tidd J, Bessant J, Pavitt K (2001) Managing innovation, integrating technological, market, and organizational change. John Wiley and Sons, Chichester
Weber W (1966) Die Reisezeit der Fahrgäste öffentlicher Verkehrsmittel in Abhängigkeit von Bahnart und Raumlage. Forschungsarbeiten des Verkehrswissenschaftlichen Instituts an der Technischen Hochschule Stuttgart, Stuttgart
Taylor CT, Hyde DJ, Barr LC (2016) Hyperloop commercial feasibility analysis: high level overview. Volpe (US Department of Transport), Cambridge
Wilkinson J (2016) A comparison of Hyperloop performances against high speed rail and air passenger transport using multi-criteria analysis: case study of the San Francisco-Los Angeles corridor. Minor thesis, Delft University of technology, Delft
Antlauf W, Bernardeau FG, Coates KC (2004) Fast track. Civil Engineering, The Magazine of the American Society of Civil Engineers 74(11):37–43
Wikipedia: https://en.wikipedia.org/wiki/Transrapid , assessed 31 October 2016
Wikipedia: https://en.wikipedia.org/wiki/Maglev , assessed 31 October 2016
TKTA: ThyssenKrupp Transrapid Australia (2008) ThyssenKrupp Transrapid Australia submission in response to East West link needs assessment report “Investing in Transport”. ThyssenKrupp Transrapid Gmbh, Kassel
Wikipedia: https://en.wikipedia.org/wiki/Gotthard_Base Tunnel , assessed 31 October 2016
Quora: https://www.quora.com/What-are-the-cost-differences-between-Maglev-and-conventional-high-speed-rail , assessed 31 October 2016
Wikipedia: https://en.wikipedia.org/wiki/Channel_Tunnel , assessed 31 October 2016
Ecorys transport, CE Delft (2005) Infrastructure expenditures and costs, Practical guidelines to calculate total infrastructure costs for five modes of transport, final report. Rotterdam. https://ec.europa.eu/transport/sites/transport/files/themes/infrastructure/studies/doc/2005_11_30_guidelines_infrastructure_report_en.pdf , assessed 31 October 2016
The World Bank (2011) Railway Reform: Toolkit for Improving Rail Sector Performance. The World Bank, Washington
Goeverden V, CD Peeters PM (2006) Suspending subsidies for public transport, its impacts on the public transport system in the Netherlands. 85th annual meeting of the Transportation Research Board, Washington
Van Goeverden CD, Janić M, Milakis D (2018) Is Hyperloop helpful in relieving the environmental burden of long-distance travel? An explorative analysis for Europe. Proceedings international conference on transport, climate change and clean air, Gif-sur-Yvette. https://www.iip.kit.edu/downloads/WCTR_SIGf2_2018_BookOfAbstracts.pdf , assessed 1 July 2018
De Decker K (2013) High Speed Trains are Killing the European Railway Network. Low-tech Magazine 15, http://www.lowtechmagazine.com/2013/12/high-speed-trains-are-killing-the-european-railway-network.html . Assessed 31 Oct 2016.
EC (2017) EU Transport in Figures. Statistical Pocketbook 2017. European Commission. Publications Office of the European Union, Luxembourg, p 164
Janić M (2007) The sustainability of air transportation: quantitative analysis and assessment. Ashgate Publishing Company, Farnham
EEC (2015) Standard Inputs for EUROCONTROL Cost-Benefit Analyses. Edition Number: 7.0 Edition Date: November 2015. European Organisation for the Safety of Air Navigation (EUROCONTROL), Brussels
UIC (2010) High speed rail: fast track to sustainability. International Union of Railways, Paris, France, http://www.hsr.ca.gov/docs/about/business_plans/BPlan_2012LibraryCh7FastTrack.pdf . Accessed 1 Apr 2017.
ICAO (2016) Carbon Emissions Calculator, https://www.icao.int/environmental-protection/CarbonOffset/Pages/default.aspx . Accessed 1 Apr 2017.
Download references
Acknowledgements
A previous version of this paper was presented at the BIVEC/GIBET Transport Research Days 2017 in Liege, Belgium.
Author information
Authors and affiliations.
Transport & Planning Department, Delft University of Technology, P.O.Box 5048, 2600 GA, Delft, the Netherlands
Kees van Goeverden, Dimitris Milakis & Milan Janic
OTB, Research for the Built Environment, Delft University of Technology, Julianalaan 134, 2628 BL, Delft, the Netherlands
Rob Konings
You can also search for this author in PubMed Google Scholar
Contributions
DM conceived the research concept and wrote the theoretical and historical background to the subject and the directions for future research. DM, MJ, KVG and RK designed the study. MJ described the HSR and APT systems and analysed the operational performance of HL. KVG analysed the financial performance of HL and contributed to the analysis of HL capacity. RK analysed the social and environmental performance of HL. All authors read and approved the final manuscript.
Corresponding author
Correspondence to Kees van Goeverden .
Ethics declarations
Competing interests.
The authors declare that they have no competing interests.
Publisher’s Note
Springer Nature remains neutral with regard to jurisdictional claims in published maps and institutional affiliations.
Rights and permissions
Open Access This article is distributed under the terms of the Creative Commons Attribution 4.0 International License ( http://creativecommons.org/licenses/by/4.0/ ), which permits unrestricted use, distribution, and reproduction in any medium, provided you give appropriate credit to the original author(s) and the source, provide a link to the Creative Commons license, and indicate if changes were made.
Reprints and permissions
About this article
Cite this article.
van Goeverden, K., Milakis, D., Janic, M. et al. Analysis and modelling of performances of the HL (Hyperloop) transport system. Eur. Transp. Res. Rev. 10 , 41 (2018). https://doi.org/10.1186/s12544-018-0312-x
Download citation
Received : 15 December 2017
Accepted : 22 August 2018
Published : 03 September 2018
DOI : https://doi.org/10.1186/s12544-018-0312-x
Share this article
Anyone you share the following link with will be able to read this content:
Sorry, a shareable link is not currently available for this article.
Provided by the Springer Nature SharedIt content-sharing initiative
- HL (Hyperloop) system
- Performances
- Long-distance transport
Overview of Factors and Methods for Analysis of Hyperloop Project
- Conference paper
- First Online: 01 February 2024
- Cite this conference paper
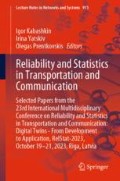
- Aleksejs Vesjolijs ORCID: orcid.org/0009-0008-2845-7498 12
Part of the book series: Lecture Notes in Networks and Systems ((LNNS,volume 913))
Included in the following conference series:
- International Conference on Reliability and Statistics in Transportation and Communication
103 Accesses
The paper is part of the research project conducted in the scope of PhD thesis “Assessment methodology for Hyperloop technology implementation for multimodal freight transportation” conducted by Aleksejs Vesjolijs. Hyperloop project is ultra-high speed transportation mode, that is envisioned in 2012 by Elon Musk. Goal of the current paper to evaluate and compare different factors, methods and innovations that have the potential to significantly impact the Hyperloop project implementation during phase of initial planning from the social, technical, economic and environmental perspectives. The following factors are covered by the research: technical feasibility, quantum factor, safety regulatory approval, social acceptance, reliability scalability, environmental sustainability, infrastructure integration, usability. Research outcomes are presented in a form of overview. The overview could be useful as an aid to decision makers such as regional and national governments, commercial transport policymakers or investors for any Hyperloop transport projects. Comparative analysis matrix is designed providing systematic view of factors and methods in evaluation of Hyperloop project.
This is a preview of subscription content, log in via an institution to check access.
Access this chapter
- Available as PDF
- Read on any device
- Instant download
- Own it forever
- Available as EPUB and PDF
- Compact, lightweight edition
- Dispatched in 3 to 5 business days
- Free shipping worldwide - see info
Tax calculation will be finalised at checkout
Purchases are for personal use only
Institutional subscriptions
European Commission: Horizon 2020 – Work Programme 2014–2015. 19 General annexes (2015). https://ec.europa.eu/research/participants/data/ref/h2020/wp/2014_2015/annexes/h2020-wp1415-annex-ga_en.pdf
Federal Register: Advancing High-Speed Rail Projects Intended for Operations Over 160 Miles Per Hour Through Domestic Sourcing Plans and Buy America Compliance (2023). https://www.federalregister.gov/documents/2023/03/22/2023-05874/advancing-high-speed-rail-projects-intended-for-operations-over-160-miles-per-hour-through-domestic
Ionita, D., Hartel, P., Pieters, W., Wieringa, R.: Current established risk assessment methodologies and tools. Technical report TR-CTIT-14-04, Centre for Telematics and Information Technology, University of Twente (2014). https://doi.org/10.13140/RG.2.2.22914.68806
Kassebi, O., Siegfried, P.: Hyperloop: the innovative logistic technology. J. Road Traff. Eng. 68 (1), 11–16 (2022). https://doi.org/10.31075/PIS.68.01.02
Kaya, A., Ozturk, R., Altin Gumussoy, C.: Usability measurement of mobile applications with System Usability Scale (SUS). In: Calisir, F., Cevikcan, E., Camgoz Akdag, H. (eds.) Industrial Engineering in the Big Data Era. LNMIE, pp. 389–400. Springer, Cham (2019). https://doi.org/10.1007/978-3-030-03317-0_32
Chapter Google Scholar
Mateu, J.M., Martínez Fernández, P., Insa Franco, R.: Setting safety foundations in the Hyperloop: a first approach to preliminary hazard analysis and safety assurance system. Saf. Sci. 142 , 105366 (2021). https://doi.org/10.1016/j.ssci.2021.105366
Article Google Scholar
Premsagar, S., Kenworthy, J.: A critical review of hyperloop (ultra-high speed rail) technology: urban and transport planning, technical, environmental, economic, and human considerations. Front. Sustain. Cities 4 , 842245 (2022). https://doi.org/10.3389/frsc.2022.842245
Rajendran, S., Harper, A.: A simulation-based approach to provide insights on Hyperloop network operations. Transp. Res. Interdisc. Perspect. 4 , 100092 (2020). https://doi.org/10.1016/j.trip.2020.100092
Sane, Y.: Multi-Criteria Analysis of the proposed Hyperloop transport project in Northern Holland. KTH Royal Institute of Technology, School of Architecture and the Built Environment, Stockholm (2020). https://kth.diva-portal.org/smash/get/diva2:1514003/FULLTEXT01.pdf
Singh, R.K., Murty, H.R., Gupta, S.K., Dikshit, A.K.: An overview of sustainability assessment methodologies. Ecol. Ind. 15 (1), 281–299 (2012). https://doi.org/10.1016/j.ecolind.2011.01.007
Tesla Homepage. https://www.tesla.com/ . Accessed 21 Nov 2023
Wetekamp, W.: Net Present Value (NPV) as a tool supporting effective project management. In: Proceedings of the 6th IEEE International Conference on Intelligent Data Acquisition and Advanced Computing Systems, Prague, Czech Republic, pp. 898–900 (2011). https://doi.org/10.1109/IDAACS.2011.6072902
Weinstock, C.B., Goodenough, J.: On system scalability. Report, Carnegie Mellon University (2018). https://kilthub.cmu.edu/articles/report/On_System_Scalability/6575879/1
White, D., Fortune, J.: Current practice in project management – an empirical study. Int. J. Proj. Manag. 20 (1), 1–11 (2002). https://doi.org/10.1016/S0263-7863(00)00029-6
Download references
Author information
Authors and affiliations.
Transport and Telecommunication Institute, Lomonosova st. 1, Riga, 1019, Latvia
Aleksejs Vesjolijs
You can also search for this author in PubMed Google Scholar
Corresponding author
Correspondence to Aleksejs Vesjolijs .
Editor information
Editors and affiliations.
Transport and Telecommunication Institute, Riga, Latvia
Igor Kabashkin
Irina Yatskiv
Vilnius Gediminas Technical University, Vilnius, Lithuania
Olegas Prentkovskis
Rights and permissions
Reprints and permissions
Copyright information
© 2024 The Author(s), under exclusive license to Springer Nature Switzerland AG
About this paper
Cite this paper.
Vesjolijs, A. (2024). Overview of Factors and Methods for Analysis of Hyperloop Project. In: Kabashkin, I., Yatskiv, I., Prentkovskis, O. (eds) Reliability and Statistics in Transportation and Communication. RelStat 2023. Lecture Notes in Networks and Systems, vol 913. Springer, Cham. https://doi.org/10.1007/978-3-031-53598-7_25
Download citation
DOI : https://doi.org/10.1007/978-3-031-53598-7_25
Published : 01 February 2024
Publisher Name : Springer, Cham
Print ISBN : 978-3-031-53597-0
Online ISBN : 978-3-031-53598-7
eBook Packages : Intelligent Technologies and Robotics Intelligent Technologies and Robotics (R0)
Share this paper
Anyone you share the following link with will be able to read this content:
Sorry, a shareable link is not currently available for this article.
Provided by the Springer Nature SharedIt content-sharing initiative
- Publish with us
Policies and ethics
- Find a journal
- Track your research
Academia.edu no longer supports Internet Explorer.
To browse Academia.edu and the wider internet faster and more securely, please take a few seconds to upgrade your browser .
Enter the email address you signed up with and we'll email you a reset link.
- We're Hiring!
- Help Center
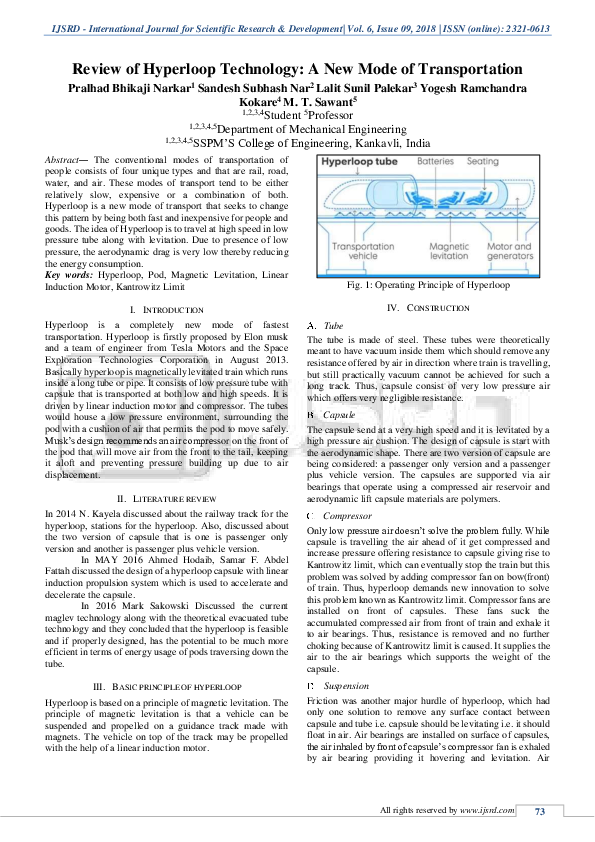
Review of Hyperloop Technology: A New Mode of Transportation | IJSRDV6I90024

2018, IJSRD - International Journal for Scientific Research and Development
— The conventional modes of transportation of people consists of four unique types and that are rail, road, water, and air. These modes of transport tend to be either relatively slow, expensive or a combination of both. Hyperloop is a new mode of transport that seeks to change this pattern by being both fast and inexpensive for people and goods. The idea of Hyperloop is to travel at high speed in low pressure tube along with levitation. Due to presence of low pressure, the aerodynamic drag is very low thereby reducing the energy consumption.
Related Papers
VIJAY SARODE
The conventional modes of transportation of people consists of four unique types and that are rail, road, water, and air. These modes of transport tend to be either relatively slow, expensive or a combination of both. Hyperloop is a new mode of transport that seeks to change this pattern by being both fast and inexpensive for people and goods. Hyperloop is a proposed mode of passenger and freight transportation that propels a capsule-like vehicle through a near-vacuum tube at more than airline speed. The pods would accelerate to cruising speed gradually using a linear electric motor and glide above their track using passive magnetic levitation or air bearings. Hyperloop consists of a low pressure tube with capsules that are transported at both low and high speeds throughout the length of the tube. The capsules are supported on a cushion of air, featuring pressurized air and aerodynamic lift. Passengers may enter and exit Hyperloop at stations located either at the ends of the tube, ...
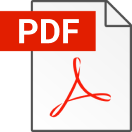
IJNTR Journal
Nextgen Research Publication
Existing conventional modes of transportation of people consists of four unique types: rail, road, water, and air. These modes of transport tend to be either relatively slow (i.e., road and water), expensive (i.e., air), or a combination of relatively slow and expensive (i.e., rail). Hyperloop is a new mode of transport that seeks to change this paradigm by being both fast and inexpensive for people and goods. Hyperloop consists of a low pressure tube with capsules that are transported at both low and high speeds throughout the length of the tube. The capsules are supported on a cushion of air, featuring pressurized air and aerodynamic lift. The capsules are accelerated via a magnetic linear accelerator affixed at various stations on the low pressure tube with rotors contained in each capsule. Passengers may enter and exit Hyperloop at stations located either at the ends of the tube, or branches along the tube length. In this study, the initial route, preliminary design, and logistics of the Hyperloop transportation system have been derived.
International Journal for Research in Applied Science and Engineering Technology
Aditya Pavadad
International Journal of Engineering Research and Technology (IJERT)
IJERT Journal
https://www.ijert.org/hyperloop-v-mode-of-transportation https://www.ijert.org/research/hyperloop-v-mode-of-transportation-IJERTV10IS020228.pdf The standard four modes of transportation which are used by commuters are railways, airways, waterways and roadways. Discussing its cost, it depends on certain factors such as debility, fragileness, distance, size, etc. and so sometimes cost increases for the individuals. Thus Elon Musk proposed the fifth mode of transportation known as Hyperloop, on white paper stating the advantage of giving twice the speed of a plane and efficiency in less time, low power consumption, cost efficient, environmental friendly as it will use solar panels and also it will not create noise. Hyperloop is a proposed mode in which a capsule like the vehicle (pod) is accelerated in an evacuated low-pressure tubes at very high speeds. These pods will be accelerated through linear induction motors, and it will also be levitated through magnetic levitation or air bearings. These pods will travel in these tubes, and passengers will be able to travel in these pods in a similar way like other conventional modes i.e. through provided hyperloop stations at prescribed locations. The two major problems are also eliminated-friction and air resistance which makes high efficiency of the pod. So it is the best replacement to high speed rail and air passenger transport.
IRJET Journal
Expected to word in the moving toward decade, Hyperloop is an attractively suspended case that articles to move voyagers between metropolitan urban areas at a rapid. To the best of the creators' information, this examination is to contemplate the Hyperloop from an operational edge by creating reproduction models. The idea of Hyperloop transportation was first presented on August 12, 2013. The arranged course runs from Los Angeles, California to Las Vegas, Nevada. As future work, the Hyperloop framework can be intended for different sets of metropolitan urban areas, and the quantity of Hyperloop cases required for efficient organize activity can be contemplated.
International Journal of Modern Trends in Engineering & Research
Shripad Chopade
IEEE Transactions on Industrial Electronics
Jawwad M. Sayeed
Samroot Samreen Wani
Sustainability
Lambros Mitropoulos
The hyperloop is an innovative land transport mode for passengers and freight that travels at ultra-high speeds. Lately, different stakeholders have been engaged in the research and development of hyperloop components. The novelty of the hyperloop necessitates certain directions to be followed toward the development and testing of its technological components as well the formation of regulations and planning processes. In this paper, we conduct a comprehensive literature review of hyperloop publications to record the current state of progress of hyperloop components, including the pod, the infrastructure, and the communication system, and identify involved EU stakeholders. Blending this information results in future directions. An online search of English-based publications was performed to finally consider 107 studies on the hyperloop and identify 81 stakeholders in the EU. The analysis shows that the hyperloop-related activities are almost equally distributed between Europe (39%) ...
RELATED TOPICS
- We're Hiring!
- Help Center
- Find new research papers in:
- Health Sciences
- Earth Sciences
- Cognitive Science
- Mathematics
- Computer Science
- Academia ©2024
Design Challenges for Hyperloop Transport Systems
Ieee account.
- Change Username/Password
- Update Address
Purchase Details
- Payment Options
- Order History
- View Purchased Documents
Profile Information
- Communications Preferences
- Profession and Education
- Technical Interests
- US & Canada: +1 800 678 4333
- Worldwide: +1 732 981 0060
- Contact & Support
- About IEEE Xplore
- Accessibility
- Terms of Use
- Nondiscrimination Policy
- Privacy & Opting Out of Cookies
A not-for-profit organization, IEEE is the world's largest technical professional organization dedicated to advancing technology for the benefit of humanity. © Copyright 2024 IEEE - All rights reserved. Use of this web site signifies your agreement to the terms and conditions.
Europe’s longest hyperloop testing center now open in Netherlands
I n the Netherlands, the longest tunnel in Europe for testing hyperloop technology is now open for business. The tunnel’s proprietors envision being able to transport people from Amsterdam to Barcelona in a matter of hours.
Following the announcement of its completed hyperloop test infrastructure, the European Hyperloop Center (EHC) is set to commence its inaugural testing phase.
This state-of-the-art facility, located near Veendam in the country’s northern region, is equipped to demonstrate all essential hyperloop technologies, including an industry-first lane-switch that can be used to test the hyperloop technologies at a speed of 47-62mph (75-100km/h).
The facility’s centerpiece is a 420-meter test tunnel comprising 34 interlinked pipes, each approximately 2.5 meters wide. Once a disused railway center, this site has transformed into a hub for exploring and validating cutting-edge innovations in transportation.
Advancing Hyperloop testing infrastructure
The Hyperloop Development Program (HDP), a public-private collaboration with over 25 partners, includes the EHC in its efforts to progress hyperloop technology.
HDP includes several hyperloop technology businesses and research institutes, including EuroTube (CH), Hardt Hyperloop (NL), Nevomo (PL), and Zeleros (ES). The Province of Groningen, the Dutch Government, and the European Commission all support the HDP financially.
EHC is an open test facility for hyperloop technology aimed at promoting the ecosystem’s technological growth. Businesses are free to use this infrastructure to test their technologies.
“This center acts as a hub for refining and validating the open specifications, allowing various stakeholders to contribute to the ongoing evolution of hyperloop technology,” said Dutch-firm Hardt Hyperloop’s website .
Together with DemoTube, a 120-meter hyperloop test facility in Switzerland owned by EuroTube, the company that develops hyperloops, EHC is a member of the European Research Infrastructure Cluster.
According to the team, the construction of the hyperloop is advanced even faster when the outcomes of the two test facilities are combined.
Hardt Hyperloop, which built and operates the facility, is scheduled to conduct initial vehicle tests in the upcoming weeks, marking a significant milestone in hyperloop technology development.
Growing interest in next-gen transportation initiatives
Elon Musk, the billionaire behind SpaceX and Tesla , popularized the hyperloop concept in 2013 when he published a paper outlining a “fifth mode of transport” connecting Los Angeles and San Francisco.
Unlike traveling by car or airline, which may take up to six hours, Musk claimed that a hyperloop tube could connect the two cities in just thirty minutes.
Global interest in the hyperloop is growing due to its technological breakthroughs. A feasibility assessment for a hyperloop pilot route has just begun in Italy. In 2026, India plans to launch a hyperloop test project.
With backing from the European Parliament, the European Commission has suggested including the hyperloop in its TEN-T program at the continental level. This program aims to improve the connectivity among the member states of the European Union.
The hyperloop has promising benefits. The infrastructure is estimated to be cheaper compared to high-speed rail. The hyperloop’s spatial integration is easier than other types of infrastructure.
Lastly, the energy use of traveling with a hyperloop is estimated to be 10 times less than traveling by car or plane, providing an energy-efficient solution for the growing transportation needs.
Despite this progress, scientists acknowledge that there is still a considerable journey ahead before the technology reaches full readiness. Passenger testing remains a distant prospect, with substantial work remaining.
As for EHC, the target for full-scale passenger operations is set for 2030, likely on a concise route spanning approximately five kilometers—such as from an airport to a city center, exemplifying the potential of this transformative mode of transportation.
Subscribe to the PwC Newsletter
Join the community, edit social preview.

Add a new code entry for this paper
Remove a code repository from this paper, mark the official implementation from paper authors, add a new evaluation result row, remove a task, add a method, remove a method, edit datasets, research on the evolution of smart meter technology.
28 Feb 2024 · Zhen Zhang · Edit social preview
Smart meter is not only a device used to measure the amount of electricity, but also a core component of the smart grid, realizing the efficient monitoring, prediction and management of power use. With an insight into the evolution of smart meter technology, I realized that this change didn't happen overnight. It has undergone a long journey from the initial mechanical electricity meters to the electronic electricity meters, and now to the highly intelligent electricity meters. Technological breakthroughs at each stage have laid the foundation for the final form of smart meters. In the era of mechanical watt-hour meters, the measurement of electric energy mainly depends on the rotation and counting of mechanical structures. The accuracy and stability of this method are affected by mechanical wear, environmental interference and other factors, and it is difficult to meet the increasing demand for power management. With the rapid development of electronic technology, the electronic electricity meter came into being. It uses electronic technology to sample and process the current and voltage, which greatly improves the accuracy and stability of the measurement. At the same time, the electronic electricity meter also has the function of remote meter reading and data processing, which has brought great convenience to the power management. However, there still have some limitations, such as the complexity of data processing and the limitation of communication capacity. It is these challenges that drive the creation of smart power meters. smart meters combine advanced technologies such as the Internet of Things, big data and cloud computing to realize the real-time monitoring, analysis and prediction of the use of power.
Code Edit Add Remove Mark official
Datasets edit.
Suggestions or feedback?
MIT News | Massachusetts Institute of Technology
- Machine learning
- Social justice
- Black holes
- Classes and programs
Departments
- Aeronautics and Astronautics
- Brain and Cognitive Sciences
- Architecture
- Political Science
- Mechanical Engineering
Centers, Labs, & Programs
- Abdul Latif Jameel Poverty Action Lab (J-PAL)
- Picower Institute for Learning and Memory
- Lincoln Laboratory
- School of Architecture + Planning
- School of Engineering
- School of Humanities, Arts, and Social Sciences
- Sloan School of Management
- School of Science
- MIT Schwarzman College of Computing
A new way to detect radiation involving cheap ceramics
Press contact :.
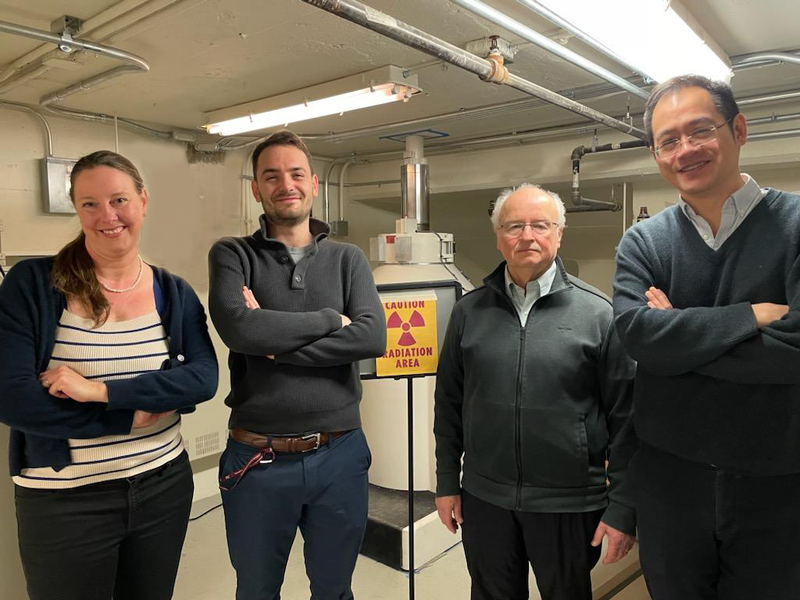
Previous image Next image
The radiation detectors used today for applications like inspecting cargo ships for smuggled nuclear materials are expensive and cannot operate in harsh environments, among other disadvantages. Now, in work funded largely by the U.S. Department of Homeland Security with early support from the U.S. Department of Energy, MIT engineers have demonstrated a fundamentally new way to detect radiation that could allow much cheaper detectors and a plethora of new applications.
They are working with Radiation Monitoring Devices , a company in Watertown, Massachusetts, to transfer the research as quickly as possible into detector products.
In a 2022 paper in Nature Materials , many of the same engineers reported for the first time how ultraviolet light can significantly improve the performance of fuel cells and other devices based on the movement of charged atoms, rather than those atoms’ constituent electrons.
In the current work, published recently in Advanced Materials , the team shows that the same concept can be extended to a new application: the detection of gamma rays emitted by the radioactive decay of nuclear materials.
“Our approach involves materials and mechanisms very different than those in presently used detectors, with potentially enormous benefits in terms of reduced cost, ability to operate under harsh conditions, and simplified processing,” says Harry L. Tuller, the R.P. Simmons Professor of Ceramics and Electronic Materials in MIT’s Department of Materials Science and Engineering (DMSE).
Tuller leads the work with key collaborators Jennifer L. M. Rupp, a former associate professor of materials science and engineering at MIT who is now a professor of electrochemical materials at Technical University Munich in Germany, and Ju Li, the Battelle Energy Alliance Professor in Nuclear Engineering and a professor of materials science and engineering. All are also affiliated with MIT’s Materials Research Laboratory
“After learning the Nature Materials work, I realized the same underlying principle should work for gamma-ray detection — in fact, may work even better than [UV] light because gamma rays are more penetrating — and proposed some experiments to Harry and Jennifer,” says Li.
Says Rupp, “Employing shorter-range gamma rays enable [us] to extend the opto-ionic to a radio-ionic effect by modulating ionic carriers and defects at material interfaces by photogenerated electronic ones.”
Other authors of the Advanced Materials paper are first author Thomas Defferriere, a DMSE postdoc, and Ahmed Sami Helal, a postdoc in MIT’s Department of Nuclear Science and Engineering.
Modifying barriers
Charge can be carried through a material in different ways. We are most familiar with the charge that is carried by the electrons that help make up an atom. Common applications include solar cells. But there are many devices — like fuel cells and lithium batteries — that depend on the motion of the charged atoms, or ions, themselves rather than just their electrons.
The materials behind applications based on the movement of ions, known as solid electrolytes, are ceramics. Ceramics, in turn, are composed of tiny crystallite grains that are compacted and fired at high temperatures to form a dense structure. The problem is that ions traveling through the material are often stymied at the boundaries between the grains.
In their 2022 paper, the MIT team showed that ultraviolet (UV) light shone on a solid electrolyte essentially causes electronic perturbations at the grain boundaries that ultimately lower the barrier that ions encounter at those boundaries. The result: “We were able to enhance the flow of the ions by a factor of three,” says Tuller, making for a much more efficient system.
Vast potential
At the time, the team was excited about the potential of applying what they’d found to different systems. In the 2022 work, the team used UV light, which is quickly absorbed very near the surface of a material. As a result, that specific technique is only effective in thin films of materials. (Fortunately, many applications of solid electrolytes involve thin films.)
Light can be thought of as particles — photons — with different wavelengths and energies. These range from very low-energy radio waves to the very high-energy gamma rays emitted by the radioactive decay of nuclear materials. Visible light — and UV light — are of intermediate energies, and fit between the two extremes.
The MIT technique reported in 2022 worked with UV light. Would it work with other wavelengths of light, potentially opening up new applications? Yes, the team found. In the current paper they show that gamma rays also modify the grain boundaries resulting in a faster flow of ions that, in turn, can be easily detected. And because the high-energy gamma rays penetrate much more deeply than UV light, “this extends the work to inexpensive bulk ceramics in addition to thin films,” says Tuller. It also allows a new application: an alternative approach to detecting nuclear materials.
Today’s state-of-the-art radiation detectors depend on a completely different mechanism than the one identified in the MIT work. They rely on signals derived from electrons and their counterparts, holes, rather than ions. But these electronic charge carriers must move comparatively great distances to the electrodes that “capture” them to create a signal. And along the way, they can be easily lost as they, for example, hit imperfections in a material. That’s why today’s detectors are made with extremely pure single crystals of material that allow an unimpeded path. They can be made with only certain materials and are difficult to process, making them expensive and hard to scale into large devices.
Using imperfections
In contrast, the new technique works because of the imperfections — grains — in the material. “The difference is that we rely on ionic currents being modulated at grain boundaries versus the state-of-the-art that relies on collecting electronic carriers from long distances,” Defferriere says.
Says Rupp, “It is remarkable that the bulk ‘grains’ of the ceramic materials tested revealed high stabilities of the chemistry and structure towards gamma rays, and solely the grain boundary regions reacted in charge redistribution of majority and minority carriers and defects.”
Comments Li, “This radiation-ionic effect is distinct from the conventional mechanisms for radiation detection where electrons or photons are collected. Here, the ionic current is being collected.”
Igor Lubomirsky, a professor in the Department of Materials and Interfaces at the Weizmann Institute of Science, Israel, who was not involved in the current work, says, “I found the approach followed by the MIT group in utilizing polycrystalline oxygen ion conductors very fruitful given the [materials’] promise for providing reliable operation under irradiation under the harsh conditions expected in nuclear reactors where such detectors often suffer from fatigue and aging. [They also] benefit from much-reduced fabrication costs.”
As a result, the MIT engineers are hopeful that their work could result in new, less expensive detectors. For example, they envision trucks loaded with cargo from container ships driving through a structure that has detectors on both sides as they leave a port. “Ideally, you’d have either an array of detectors or a very large detector, and that’s where [today’s detectors] really don’t scale very well,” Tuller says.
Another potential application involves accessing geothermal energy, or the extreme heat below our feet that is being explored as a carbon-free alternative to fossil fuels. Ceramic sensors at the ends of drill bits could detect pockets of heat — radiation — to drill toward. Ceramics can easily withstand extreme temperatures of more than 800 degrees Fahrenheit and the extreme pressures found deep below the Earth’s surface.
The team is excited about additional applications for their work. “This was a demonstration of principle with just one material,” says Tuller, “but there are thousands of other materials good at conducting ions.”
Concludes Defferriere: “It’s the start of a journey on the development of the technology, so there’s a lot to do and a lot to discover.”
This work is currently supported by the U.S. Department of Homeland Security, Countering Weapons of Mass Destruction Office. This support does not constitute an express or implied endorsement on the part of the government. It was also funded by the U.S. Defense Threat Reduction Agency.
Share this news article on:
Related links.
- Harry Tuller
- Tuller Research Group
- Materials Research Laboratory
Related Topics
- Nuclear security and policy
- Materials science and engineering
- Nuclear science and engineering
- Department of Energy (DoE)
Related Articles
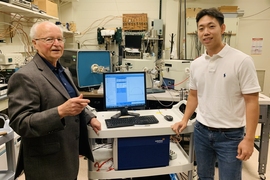
A simple way to significantly increase lifetimes of fuel cells and other devices
Harry Tuller honored for career advancing solid-state chemistry and electrochemistry
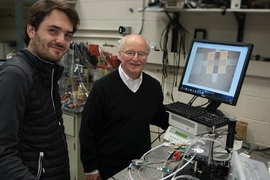
Light could boost performance of fuel cells, lithium batteries, and other devices
Previous item Next item
More MIT News
A biomedical engineer pivots from human movement to women’s health
Read full story →
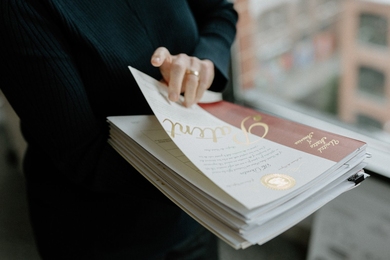
MIT tops among single-campus universities in US patents granted
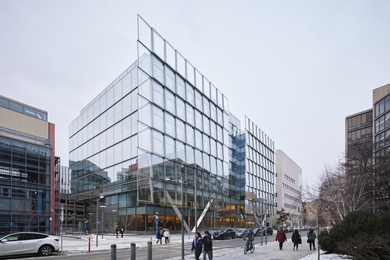
A crossroads for computing at MIT
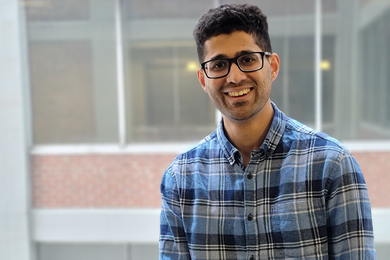
Growing our donated organ supply
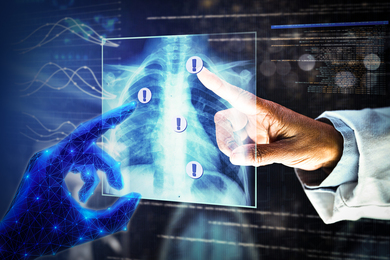
New AI method captures uncertainty in medical images
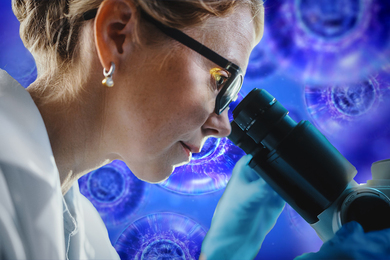
Improving drug development with a vast map of the immune system
- More news on MIT News homepage →
Massachusetts Institute of Technology 77 Massachusetts Avenue, Cambridge, MA, USA
- Map (opens in new window)
- Events (opens in new window)
- People (opens in new window)
- Careers (opens in new window)
- Accessibility
- Social Media Hub
- MIT on Facebook
- MIT on YouTube
- MIT on Instagram
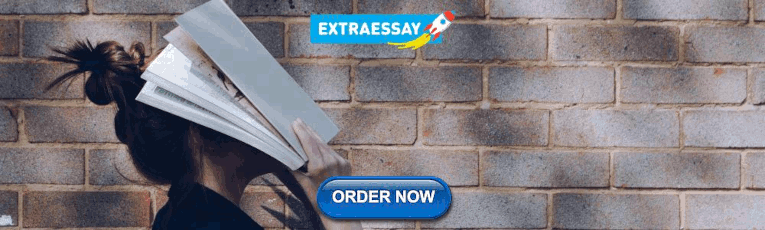
IMAGES
VIDEO
COMMENTS
Tel.: +39-0332-78-6041. Abstract: Hyperloop is a proposed very high-speed ground transportation system for both passenger. and freight that has the potential to be revolutionary, and which has ...
ABSTRACT. The Hyperloop concept, pod speed competitions and current project developments have recently attracted much publicity. In this paper the transport technology of the vacuumed tube transport project Hyperloop is assessed through a system analysis of its principal aims, functional design, transport capacity and demand in comparison with existing commercial airlines, high-speed rail, and ...
Hyperloop is a proposed very high-speed ground transportation system for both passenger and freight that has the potential to be revolutionary, and which has attracted much attention in the last few years. The concept was introduced in its modern form relatively recently, yet substantial progress has been made in the past years, with research and development taking place globally, from several ...
Companies globally have been trying to alter existing transportation systems to maximize public transport benefits, especially in the sector of high-speed (HS) ground transportation. The latest invention of such is Elon Musk's ultra-high-speed rail (UHSR), hyperloop. This technology enables a rail service to operate at a potential speed of 1,200 km/h. Hyperloop's ambitious speed goal has the ...
This paper provides an overview of hyperloop technologies, including a brief discussion of key components of a hyperloop system that determine the electric power requirements as a function of time. At-scale hyperloop systems do not yet exist, so a model was used to generate a set of load profiles for conceptual hyperloop realizations at four locations in the United States: two systems in ...
The hyperloop is an innovative land transport mode for passengers and freight that travels at ultra-high speeds. Lately, different stakeholders have been engaged in the research and development of hyperloop components. The novelty of the hyperloop necessitates certain directions to be followed toward the development and testing of its technological components as well the formation of ...
This study introduces a novel methodology based on an extensive mathematical analysis, performed from basic electromagnetic principles, with an optimized cost for a magnetic levitation Hyperloop system. This new approach uses both permanent magnets and electromagnets to levitate, propel, and control a pod. The electrodynamic suspension system is emulated as a small pod attached with permanent ...
Hyperloop Academic Research: A Systematic Review and a Taxonomy of Issues. K. Gkoumas. Published in Applied Sciences 26 June 2021. Engineering, Business, Environmental Science. Hyperloop is a proposed very high-speed ground transportation system for both passenger and freight that has the potential to be revolutionary, and which has attracted ...
The Hyperloop is a proposed novel mode of high-speed transportation that uses magnetically levitated pods, propelled by a linear synchronous motor in a partially evacuated environment to transport passengers. It is being commercialized on multiple routes across the world with different operating speeds: Mumbai-Pune (India-360 km/h), Dubai-Abu Dhabi (UAE-750 km/h), Chicago-Pittsburgh (USA ...
This system uses a. capsule whi ch travels inside the tube at th e speed of 12 20. kmph, which will cover the distance of 561km in approx. 30. minutes. This ultra-high sp eed transportation system ...
This paper is an analysis of the current state of the Hyperloop technology, containing studies for eventual development projects, scheduled for either a medium or a long term. This requires considerable research work, mainly basing on Internet sources. All the information reflects the current technological status for 2019.
High-speed rail (HSR) communication has always been an attractive research topic with the continuous progress of transportation systems and communication technologies. Recently, Hyperloop has emerged as a candidate for very high-speed transportation systems. Because of its outstanding potential, Hyperloop can usher in a new transportation era with several attractive features. Developing ...
Hyperloop (HL) is presented as an efficient alternative of HSR (High Speed Rail) and APT (Air Passenger Transport) systems for long-distance passenger transport. This paper explores the performances of HL and compares these performances to HSR and APT. The following performances of the HL system are analytically modeled and compared to HSR and APT: (i) operational performance; (ii) financial ...
Abstract and Figures. Abstract-This paper provides an overview of hyperloop technologies, including a brief discussion of key components of a hyperloop system that determine the electric power ...
The Hyperloop concept, pod speed competitions and current project developments have recently attracted much publicity. In this paper the transport technology of the vacuumed tube transport project ...
Figure 1. Evolution of Hyperloop academic research. Figure 1 shows an overview of the results, which are destined to increase in 2021. After After this this step, step, an an analysis analysis of of all all abstracts abstracts (and (and in in case case of doubt, of doubt, of the of the full full paper) paper) took took place, place, and and the ...
This review paper examines the appropriateness of a hyperloop line between Mumbai and Pune in India, examining, in particular, its potential economic implications and impact on people. This assessment builds on an earlier in-depth examination by the authors of the key urban and transport planning, technical, environmental, economic and human factors surrounding the hyperloop technology.
Hyperloop is a break-through technology for super-fast-speed land transportation introduced in 2013, proposed by Tesla and Space X [].The main goal of the research is to evaluate and compare different factors, methods and innovations that have the potential to significantly impact the hyperloop implementation.
The Hyperloop passenger plus vehicle capsule overall interior weight is expected to be near 6,000 lb (2,700 kg) including the seats, restraint systems, interior and door panels, luggage compartments, and entertainment displays. The overall cost of the interior components is targeted to be no more than $185,000.
IJSRD - International Journal for Scientific Research & Development| Vol. 6, Issue 09, 2018 | ISSN (online): 2321-0613 Review of Hyperloop Technology: A New Mode of Transportation Pralhad Bhikaji Narkar1 Sandesh Subhash Nar2 Lalit Sunil Palekar3 Yogesh Ramchandra Kokare4 M. T. Sawant5 1,2,3,4 Student 5Professor 1,2,3,4,5 Department of ...
This review paper aims to provide a comprehensive overview of the Hyperloop transportation system, covering its conceptualization, design, technical aspects, challenges, and potential implications ...
Abstract: Hyperloop technology testing and development can be the center of attention in the future as the concept is appealing to some scientific, industry, and business communities alike. Research and development are currently taking place in some research labs around the globe with small-scale prototypes being tested. While the technology appears to have advantages over some other modes of ...
HDP includes several hyperloop technology businesses and research institutes, including EuroTube (CH), Hardt Hyperloop (NL), Nevomo (PL), and Zeleros (ES). ... popularized the hyperloop concept in ...
Research on the evolution of smart meter technology. 28 Feb 2024 · Zhen Zhang ·. Edit social preview. Smart meter is not only a device used to measure the amount of electricity, but also a core component of the smart grid, realizing the efficient monitoring, prediction and management of power use. With an insight into the evolution of smart ...
The hyperloop is an innovative land transport mode for passengers and freight that travels at ultra-high speeds. Lately, different stakeholders have been engaged in the research and development of ...
In the current paper they show that gamma rays also modify the grain boundaries resulting in a faster flow of ions that, in turn, can be easily detected. And because the high-energy gamma rays penetrate much more deeply than UV light, "this extends the work to inexpensive bulk ceramics in addition to thin films," says Tuller.