Neurobiology of Depression
- First Online: 03 February 2022
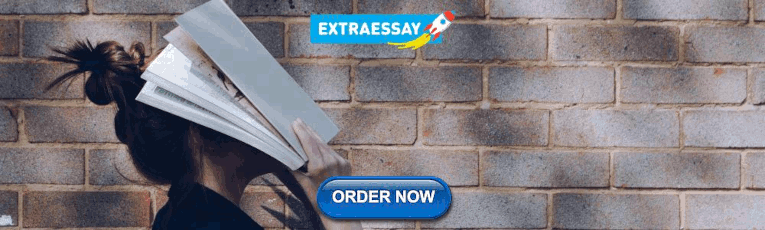
Cite this chapter
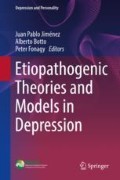
- Hernán Silva 7
Part of the book series: Depression and Personality ((DP))
686 Accesses
Hypotheses about the pathophysiology of depression have evolved over time. This chapter covers the most important findings in this regard. First, the classical monoamine hypothesis posited that depression is caused by an alteration in levels of one or more of the monoamines: serotonin, norepinephrine, and dopamine. More recently, research on the glutamatergic system has aroused great interest by examining the mechanism of action of ketamine, an N-methyl-D-aspartic acid (NMDA) receptor antagonist. Likewise, stressful life events can precipitate depressive episodes in vulnerable individuals. Abnormalities in the HPA axis have been associated with a hyperactive response to stress in depressed patients (the diathesis-stress model). Increased levels of inflammatory markers have been found in patients with depression and anti-inflammatory agents are being studied as antidepressants. Reduced production of BDNF and neuroplasticity can lead to depression. These pathophysiological mechanisms are reciprocally connected with each other. Major Depression is a heterogeneous entity and a variety of biological mechanisms may be involved.
- Pathophysiology
- Monoamine hypothesis
- Inflammation
- Neurogenesis
This is a preview of subscription content, log in via an institution to check access.
Access this chapter
- Available as PDF
- Read on any device
- Instant download
- Own it forever
- Available as EPUB and PDF
- Compact, lightweight edition
- Dispatched in 3 to 5 business days
- Free shipping worldwide - see info
- Durable hardcover edition
Tax calculation will be finalised at checkout
Purchases are for personal use only
Institutional subscriptions
Amidfar, M., Woelfer, M., Réus, G. Z., Quevedo, J., Walter, M., & Kim, Y. K. (2019). The role of NMDA receptor in neurobiology and treatment of major depressive disorder: Evidence from translational research. Progress in Neuro-Psychopharmacology & Biological Psychiatry, 94 (Aug 30), 109668. https://doi.org/10.1016/j.pnpbp.2019.109668
Article Google Scholar
Bao, A. M., & Swaab, D. F. (2018). The human hypothalamus in mood disorders: The HPA axis in the center. IBRO Reports, 6 (Dec 14), 45–53. https://doi.org/10.1016/j.ibror.2018.11.008
Article PubMed PubMed Central Google Scholar
Bousman, C. A., Forbes, M., Jayaram, M., Eyre, H., Reynolds, C. F., Berk, M., Hopwood, M., & Ng, C. (2017). Antidepressant prescribing in the precision medicine era: A prescriber’s primer on pharmacogenetics tools. BMC Psychiatry, 17 (1), 60. https://doi.org/10.1186/s12888-017-1230-5
Casey, B. J., Craddock, N., Cuthbert, B. N., Hyman, S. E., Lee, F. S., & Ressler, K. J. (2013). DSM-5 and RDoC: Progress in psychiatry research? Nature Reviews Neuroscience. Nov, 14 (11), 810–814. https://doi.org/10.1038/nrn3621
Dantzer, R., O’Connor, J. C., Freund, G., Johnson, R. W., & Kelley, K. W. (2007). From information to sickness and depression: When the immune system subjugates the brain. Nature Reviews. Neuroscience, 9 , 45–56.
Google Scholar
Dean, J., & Keshavan, M. (2017). The neurobiology of depression: An integrated view. Asian Journal of Psychiatry. Jun, 27 , 101–111. https://doi.org/10.1016/j.ajp.2017.01.025
Ding, Y., & Dai, J. (2019). Advance in stress for depressive disorder. Advances in Experimental Medicine and Biology, 1180 , 147–178. https://doi.org/10.1007/978-981-32-9271-0_8
Article PubMed Google Scholar
Duman, R. S. (2009). Neuronal damage and protection in the pathophysiology and treatment of psychiatric illness: Stress and depression. Dialogues in Clinical Neuroscience, 11 (3), 239–255.
Duman, R. S., Shinohara, R., Fogaça, M. V., & Hare, B. (2019). Neurobiology of rapid-acting antidepressants: Convergent effects on GluA1-synaptic function. Molecular Psychiatry, 2019 Dec, 24 (12), 1816–1832. https://doi.org/10.1038/s41380-019-0400-x
Ferrari, F., & Villa, R. F. (2017). The neurobiology of depression: An integrated overview from biological theories to clinical evidence. Molecular Neurobiology. Sep, 54 (7), 4847–4865. https://doi.org/10.1007/s12035-016-0032-y
Gold, P. W. (2015). The organization of the stress system and its dysregulation in depressive illness. Molecular Psychiatry, Feb, 20 (1), 32–47. https://doi.org/10.1038/mp.2014.163
Gould, E., Tanapat, P., Rydel, T., & Hastings, N. (2000). Regulation of hippocampal neurogenesis in adulthood. Biological Psychiatry, 48 , 715–720.
Iadarola, N. D., Niciu, M. J., Richards, E. M., Vande Voort, J. L., Ballard, E. D., Lundin, N. B., Nugent, A. C., Machado-Vieira, R., & Zarate, C. A., Jr. (2015). Ketamine and other N-methyl-D-aspartate receptor antagonists in the treatment of depression: A perspective review. Therapeutic Advances in Chronic Disease. May, 6 (3), 97–114. https://doi.org/10.1177/2040622315579059
Jesulola, E., Micalos, P., & Baguley, I. J. (2018). Understanding the pathophysiology of depression: From monoamines to the neurogenesis hypothesis model - are we there yet? Behavioural Brain Research, 341 (Apr 2), 79–90. https://doi.org/10.1016/j.bbr.2017.12.025
Kanter, J. W., Busch, A. M., Weeks, C. E., & Landes, S. J. (2008). The nature of clinical depression: Symptoms, syndromes, and behavior analysis. Behavior Analyst, 31 (1), 1–21.
Lamers, F., Vogelzangs, N., Merikangas, K. R., de Jonge, P., Beekman, A. T., & Penninx, B. W. (2013). Evidence for a differential role of HPA-axis function, inflammation and metabolic syndrome in melancholic versus atypical depression. Molecular Psychiatry. Jun, 18 (6), 692–699. https://doi.org/10.1038/mp.2012.144
Leonard, B. E. (2001). Stress, norepinephrine and depression. Journal of Psychiatry & Neuroscience, 26 (Suppl), S11.
Liang, S., Wu, X., Hu, X., Wang, T., & Jin, F. (2018). Recognizing depression from the microbiota-gut-brain axis. International Journal of Molecular Sciences, 19 (6). https://doi.org/10.3390/ijms19061592
Liu, C. H., Zhang, G. Z., Li, B., Li, M., Woelfer, M., Walter, M., & Wang, L. (2019). Role of inflammation in depression relapse. Journal of Neuroinflammation . Apr 17 , 16 (1), 90. https://doi.org/10.1186/s12974-019-1475-7
Machado-Vieira, R., Salvadore, G., Diaz Granados, N., Ibrahim, L., Latov, D., Wheeler-Castillo, C., Baumann, J., Henter, I. D., & Zarate, C. A. (2010). New therapeutic targets for mood disorders. The Scientific World Journal, 10 , 713–726. https://doi.org/10.1100/tsw.2010.65
Miller, H. L., Delgado, P. L., Salomon, R. M., Berman, R., Krystal, J. H., Heninger, G. R., et al. (1996). Clinical and biochemical effects of catecholamine depletion on antidepressant-induced remission of depression. Archives of General Psychiatry, 53 , 117–128.
Monteleone, P., Serritella, C., Martiadis, V., & Maj, M. (2008). Decreased levels of serum brain-derived neurotrophic factor in both depressed and euthymic patients with unipolar depression and in euthymic patients with bipolar I and II disorders. Bipolar Disorders, 10 (1), 95–100. https://doi.org/10.1111/j.1399-5618.2008.00459.x
Pitsillou, E., Bresnehan, S. M., Kagarakis, E. A., Wijoyo, S. J., Liang, J., Hung, A., & Karagiannis, T. C. (2020). The cellular and molecular basis of major depressive disorder: Towards a unified model for understanding clinical depression. Molecular Biology Reports. Jan, 47 (1), 753–770. https://doi.org/10.1007/s11033-019-05129-3
Raison, C. L., Capuron, L., & Miller, A. (2006). Cytokines sing the blues: Inflammation and the pathogenesis of depression. Trends in Immunology, 27 , 24–31.
Ruhé, H. G., Mason, N. S., & Schene, A. H. (2007). Mood is indirectly related to serotonin, norepinephrine and dopamine levels in humans: A meta-analysis of monoamine depletion studies. Molecular Psychiatry, 12 , 331–359.
Sanacora, G., Treccani, G., & Popoli, M. (2012). Towards a glutamate hypothesis of depression: An emerging frontier of neuropsychopharmacology for mood disorders. Neuropharmacology, 62 , 63–77. https://doi.org/10.1016/j.neuropharm.2011.07.036
Steptoe, A., Hamer, M., & Chida, Y. (2007). The effects of acute psychological stress on circulating inflammatory factors in humans: A review and meta-analysis. Brain, Behavior, and Immunity, 21 (7), 901–912.
Villas Boas, G. R., Boerngen de Lacerda, R., Paes, M. M., Gubert, P., Almeida, W. L. D. C., Rescia, V. C., De Carvalho, P. M. G., De Carvalho, A. A. V., & Oesterreich, S. A. (2019). Molecular aspects of depression: A review from neurobiology to treatment. European Journal of Pharmacology . May 15 , 851 , 99–121. https://doi.org/10.1016/j.ejphar.2019.02.024
Willner, P., Scheel-Krüger, J., & Belzung, C. (2013). The neurobiology of depression and antidepressant action. Neuroscience and Biobehavioral Reviews, 37 (10 Pt 1), 2331–2371. Dec. https://doi.org/10.1016/j.neubiorev.2012.12.007
Woelfer, M., Kasties, V., Kahlfuss, S., & Walter, M. (2019). The role of depressive subtypes within the neuroinflammation hypothesis of major depressive disorder. Neuroscience, 403 , 93–110. https://doi.org/10.1016/j.neuroscience.2018.03.034 . Apr 1.
Wohleb, E. S., Franklin, T., Iwata, M., & Duman, R. S. (2016). Integrating neuroimmune systems in the neurobiology of depression. Nature Reviews Neuroscience. Aug, 17 (8), 497–511. https://doi.org/10.1038/nrn.2016.69
Download references
Author information
Authors and affiliations.
Department of Psychiatry, North Campus, Faculty of Medicine, University of Chile, Santiago, Chile
Hernán Silva
You can also search for this author in PubMed Google Scholar
Editor information
Editors and affiliations.
Department of Psychiatry and Mental Health East, Faculty of Medicine, University of Chile, Millennium Institute for Research in Depression and Personality (MIDAP), Santiago, RM, Chile
Juan Pablo Jiménez
Alberto Botto
Research Department of Clinical Educational and Health Psychology, University College London, London, UK
Peter Fonagy
Rights and permissions
Reprints and permissions
Copyright information
© 2021 Springer Nature Switzerland AG
About this chapter
Silva, H. (2021). Neurobiology of Depression. In: Jiménez, J.P., Botto, A., Fonagy, P. (eds) Etiopathogenic Theories and Models in Depression. Depression and Personality. Springer, Cham. https://doi.org/10.1007/978-3-030-77329-8_8
Download citation
DOI : https://doi.org/10.1007/978-3-030-77329-8_8
Published : 03 February 2022
Publisher Name : Springer, Cham
Print ISBN : 978-3-030-77328-1
Online ISBN : 978-3-030-77329-8
eBook Packages : Behavioral Science and Psychology Behavioral Science and Psychology (R0)
Share this chapter
Anyone you share the following link with will be able to read this content:
Sorry, a shareable link is not currently available for this article.
Provided by the Springer Nature SharedIt content-sharing initiative
- Publish with us
Policies and ethics
- Find a journal
- Track your research
The current state of the neurogenic theory of depression and anxiety
Affiliations.
- 1 Department of Psychiatry, Columbia University, New York, NY, USA, Division of Integrative Neuroscience, New York State Psychiatric Institute, New York, NY, USA.
- 2 Department of Neuroscience, Columbia University, New York, NY, USA, Department of Pharmacology, Columbia University, New York, NY, USA, Department of Psychiatry, Columbia University, New York, NY, USA, Division of Integrative Neuroscience, New York State Psychiatric Institute, New York, NY, USA. Electronic address: [email protected].
- PMID: 25240202
- PMCID: PMC4293252
- DOI: 10.1016/j.conb.2014.08.012
Newborn neurons are continuously added to the adult hippocampus. Early studies found that adult neurogenesis is impaired in models of depression and anxiety and accelerated by antidepressant treatment. This led to the theory that depression results from impaired adult neurogenesis and restoration of adult neurogenesis leads to recovery. Follow up studies yielded a complex body of often inconsistent results, and the veracity of this theory is uncertain. We propose five criteria for acceptance of this theory, we review the recent evidence for each criterion, and we draw the following conclusions: Diverse animal models of depression and anxiety have impaired neurogenesis. Neurogenesis is consistently boosted by antidepressants in animal models only when animals are stressed. Ablation of neurogenesis in animal models impairs cognitive functions relevant to depression, but only a minority of studies find that ablation causes depression or anxiety. Recent human neuroimaging and postmortem studies are consistent with the neurogenic theory, but they are indirect. Finally, a novel drug developed based on the neurogenic theory is promising in animal models.
Copyright © 2014 Elsevier Ltd. All rights reserved.
Publication types
- Research Support, N.I.H., Extramural
- Research Support, Non-U.S. Gov't
- Antidepressive Agents / therapeutic use
- Anxiety / drug therapy
- Anxiety / pathology*
- Depression / drug therapy
- Depression / pathology*
- Disease Models, Animal
- Hippocampus / pathology
- Neurogenesis / drug effects
- Neurogenesis / physiology*
- Neurons / drug effects
- Neurons / physiology*
- Antidepressive Agents
Grants and funding
- R25 MH086466/MH/NIMH NIH HHS/United States
- R25 MH086466-03/MH/NIMH NIH HHS/United States
- R01 AG043688/AG/NIA NIH HHS/United States
- R01 NS081203/NS/NINDS NIH HHS/United States
- R37 MH068542/MH/NIMH NIH HHS/United States
- R01NS081203-01A1/NS/NINDS NIH HHS/United States
Click through the PLOS taxonomy to find articles in your field.
For more information about PLOS Subject Areas, click here .
Loading metrics
Open Access
Peer-reviewed
Research Article
The reduction of adult neurogenesis in depression impairs the retrieval of new as well as remote episodic memory
Roles Data curation, Formal analysis, Investigation, Methodology, Project administration, Validation, Visualization, Writing – original draft, Writing – review & editing
Affiliations Institute for Neural Computation, Ruhr University Bochum, Bochum, Germany, Mercator Research Group “Structure of Memory”, Ruhr University Bochum, Bochum, Germany, Faculty of Psychology, Ruhr University Bochum, Bochum, Germany
Roles Investigation, Methodology, Writing – review & editing
Affiliation St. Elisabeth Hospital, Gütersloh, Germany
Roles Conceptualization, Funding acquisition, Supervision, Writing – review & editing
* E-mail: [email protected]
Affiliations Institute for Neural Computation, Ruhr University Bochum, Bochum, Germany, Mercator Research Group “Structure of Memory”, Ruhr University Bochum, Bochum, Germany
- Jing Fang,
- Selver Demic,
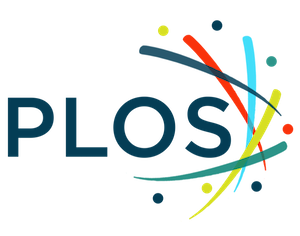
- Published: June 7, 2018
- https://doi.org/10.1371/journal.pone.0198406
- Reader Comments
Major depressive disorder (MDD) is associated with an impairment of episodic memory, but the mechanisms underlying this deficit remain unclear. Animal models of MDD find impaired adult neurogenesis (AN) in the dentate gyrus (DG), and AN in DG has been suggested to play a critical role in reducing the interference between overlapping memories through pattern separation. Here, we study the effect of reduced AN in MDD on the accuracy of episodic memory using computational modeling. We focus on how memory is affected when periods with a normal rate of AN (asymptomatic states) alternate with periods with a low rate (depressive episodes), which has never been studied before. Also, unlike previous models of adult neurogenesis, which consider memories as static patterns, we model episodic memory as sequences of neural activity patterns. In our model, AN adds additional random components to the memory patterns, which results in the decorrelation of similar patterns. Consistent with previous studies, higher rates of AN lead to higher memory accuracy in our model, which implies that memories stored in the depressive state are impaired. Intriguingly, our model makes the novel prediction that memories stored in an earlier asymptomatic state are also impaired by a later depressive episode. This retrograde effect exacerbates with increased duration of the depressive episode. Finally, pattern separation at the sensory processing stage does not improve, but rather worsens, the accuracy of episodic memory retrieval, suggesting an explanation for why AN is found in brain areas serving memory rather than sensory function. In conclusion, while cognitive retrieval biases might contribute to episodic memory deficits in MDD, our model suggests a mechanistic explanation that affects all episodic memories, regardless of emotional relevance.
Citation: Fang J, Demic S, Cheng S (2018) The reduction of adult neurogenesis in depression impairs the retrieval of new as well as remote episodic memory. PLoS ONE 13(6): e0198406. https://doi.org/10.1371/journal.pone.0198406
Editor: Judith Homberg, Radboud University Medical Centre, NETHERLANDS
Received: October 10, 2017; Accepted: May 20, 2018; Published: June 7, 2018
Copyright: © 2018 Fang et al. This is an open access article distributed under the terms of the Creative Commons Attribution License , which permits unrestricted use, distribution, and reproduction in any medium, provided the original author and source are credited.
Data Availability: All relevant data are within the paper and its Supporting Information files.
Funding: This work was supported by the German Research Foundation (DFG) through the SFB 874 project B2, grant 01GQ1506 and a grant from the Stiftung Mercator. The funders had no role in study design, data collection and analysis, decision to publish, or preparation of the manuscript.
Competing interests: The authors have declared that no competing interests exist.
Introduction
Major depressive disorder (MDD) is the most common mood disorder, estimated to affect 20% of the population at some point of a person’s lifetime [ 1 – 3 ]. MDD is characterized by a constellation of behavioural, emotional and cognitive symptoms, especially in the domain of memory [ 4 ]. Numerous studies have reported a selective impairment of episodic memory during depressive episodes [ 5 – 8 ]. Some studies even find an almost linear relationship between scores on a depression rating scale and episodic memory performance [ 9 , 10 ]. Unlike episodic memory, however, semantic memory, the other type of declarative memory, is relatively intact in MDD patients [ 11 , 12 ].
The mechanisms underlying MDD are not understood. The neurogenic theory of depression suggests that impaired adult neurogenesis (AN) in the dentate gyrus (DG) triggers depression and that restoration of AN leads to recovery [ 13 ]. AN refers to the process that generates new neurons beyond development in adulthood. It occurs in only two regions of the mammalian brain, one of which is the DG. A number of experimental studies have observed a reduction of AN in animal models of MDD [ 14 – 18 ]. While there are no direct measurements of AN in brains of MDD patients, both post-mortem [ 19 ] and high-resolution MRI volumetric [ 20 , 21 ] studies consistently find smaller DG sizes in subjects who had suffered or were suffering from MDD. In addition, animal studies indicate that the rate of AN can be increased by antidepressant treatment [ 22 – 24 ] and ablating AN suppresses the antidepressant effect of the drug [ 14 , 25 ]. However, the clear picture painted by these studies is complicated by findings that even a complete reduction of AN [ 25 ] does not produce the behavioural symptoms of MDD, see [ 26 ] for a review. Nevertheless, even though the role of AN in the etiology of MDD remains uncertain, the evidence strongly suggests that there is a correlation between MDD and AN in DG.
The DG is a subregion of the hippocampus, which is heavily involved in the storage and retrieval of episodic memory [ 27 – 29 ]. Marr [ 30 ] suggested that memories are stored in an associative network that is implemented in the recurrent connections of hippocampal CA3. Computational studies suggest that memory patterns in CA3 have to be uncorrelated to avoid interference between memories. Since sensory inputs are highly correlated, the hippocampal network has to pre-proccess these input patterns to reduce the correlations before they can be stored in CA3 [ 31 ]. This process is called pattern separation and the DG, which receives inputs from the entorhinal cortex (EC) and sends direct projections to CA3, has been suggested to be especially suitable for this purpose [ 30 , 32 – 34 ]. There is mounting empirical support for the hypothesis that AN in DG plays a role in minimizing interference between overlapping memories. Animals with AN impairment show a deficit in spatial discrimination [ 35 – 37 ] and in learning overlapping odour pair discriminations [ 38 ]. On the other hand, increasing AN improves pattern separation [ 39 ]. An fMRI study in humans also shows that the presentation of objects that are very similar, but not identical, to previously learned objects increases BOLD activity in human DG/CA3 [ 40 ]. Linking MDD, AN, and pattern separation together, recent studies in humans found a negative correlation between depression scores and pattern separation performance [ 41 , 42 ]. Déry et al. also find that the memory deficit in depression is selective for a neurogenesis-dependent task, and does not occur in other hippocampus-dependent control tasks [ 41 ].
In contrast to the abundance of experimental and clinical studies on the link between MDD and cognitive deficits, there are few modelling studies on this topic. One example is the study by Becker et al., who proposed a functional cluster hypothesis in their theoretical model by which cells born at a particular time in the DG encode a context that binds together all memories formed in that context [ 43 ]. An AN deficit then causes deficits in contextual memory. By contrast, the vast majority of computational studies focus on the broader question of how AN contributes to normal learning and memory, see [ 44 ] for a review. AN is implemented either by replacing trained cells with new naïve cells or generate additional new cells. In simple feedforward architectures, neural replacement improves the encoding of new memories at the cost of losing previously stored memories [ 45 – 48 ]. By contrast, adding new neurons to the network can help avoid catastrophic interference [ 49 ] and can preserve old memories as well as store and retrieve new memories [ 50 ]. Aimone et al. emphasizes the role of newborn immature granule cells which are more broadly tuned to a wide range of inputs [ 51 ]. Their model suggests that immature neurons increase the similarity between contemporaneous events, but once they are mature, they separate events that occur in different time periods. Nonetheless, these computational studies do not account for the specific episodic memory deficits in MDD.
Finally, little is known about how dynamic changes in the rate of AN might affect episodic memories. The time course of MDD is highly dynamic [ 52 , 53 ] and involves transitions between depressive episodes, when the rate of AN is putatively low, and asymptomatic states, when the rate of AN is putatively higher. Although memory deficits in depressive patients have been reported in various episodic memory tasks, these studies generally examine the memories both formed and retrieved in the depressive state. The accuracy of memories formed during asymptomatic states and retrieved during depressive episodes, or vice versa, has not been studied using controlled experiments. Note that this cannot be achieved by asking depressive patients to recall auto-biographical memories stored in a previous asymptomatic state, since not all auto-biographical memory can be considered episodic memory [ 28 , 54 ].
In this study, we develop a computational model that accounts for episodic memory deficits in MDD by assuming that MDD leads to a reduction in DG AN, which in turn leads an impairment in pattern separation, which eventually impairs episodic memory retrieval. Unlike previous models of adult neurogenesis, which consider memories as static patterns, we model episodic memory as sequences of neural activity patterns. Also, we examine for the first time how episodic memories are affected by the dynamics of MDD. To simulate this dynamics, the model differentiates an asymptomatic state with a normal rate of AN and a depressive state with reduced rate of AN. We compare the retrieval of memories stored and retrieved in the same state as well as memories stored in one state and retrieved in another. We find that pattern separation indeed improves episodic memory retrieval as well as its robustness to the retrieval noise. Retrieval performance is significantly worse for memories stored and retrieved in the depressive state as compared to the asymptomatic state. Interestingly, our model predicts an retrograde effect of MDD on memories formed in an earlier asymptomatic state. This effect is a novel prediction of our model, which has not been previously reported by any study, experimental or computational.
Memory model
To study episodic memory storage and retrieval, we adopted a model that we proposed and studied in earlier work [ 55 ]. The model consists of three systems (the perceptual, semantic and episodic system), which are arranged hierarchically ( Fig 1A ). This model assumes that episodic memories are represented as sequences of activation patterns, which are stored in the hippocampus [ 28 , 29 , 56 ]. These activation patterns are the outputs of a semantic representation network in neocortex, which generates low dimensional semantic representations of high dimensional sensory input. In other word, episodic memory in the model is defined as sequences of semantic representations.
- PPT PowerPoint slide
- PNG larger image
- TIFF original image
A. The relationship between systems involved episodic memory. B. Example of the input stimuli. Top: 300 × 300 black-and-white pixels; bottom: pattern scaled down to 30 × 30 greyscale pixels. C. Hierarchical network of slow feature analysis (SFA) as a model of the semantic system. The dots in each layer symbolize SFA nodes. The grey patches indicate the receptive field of each node, partially overlapping with the neighbouring nodes’ receptive fields. As an ensemble nodes in a given layer cover the full input space. Each node performs a number of processing steps as visualized on the right hand side. The activity in the top layer is taken as the output of the semantic system in our memory model. D. Example of the output of the semantic representation layer. The object in the input sequence i moves along the trajectory (yellow arrow) and rotates by 360 degrees (indicated by black arrows). Shown on the right are the four slowest features calculated by the SFA-network. The feature values at time t , y i , t (dashed line), form a semantic (more abstract) representation of the input. E. Sequence storage network (see main text in Methods for details).
https://doi.org/10.1371/journal.pone.0198406.g001
Input stimuli.
Test sequences were generated using a different statistics to ensure that our results are not selective to the specific input statistics used during training. In the test sequences, the object moved along a random walk trajectory, where the object can translate horizontally and vertically in each time step. The step sizes in the two directions were drawn independently from a normal distribution v ∼ N (5, 2.2). If a step would have taken the object beyond the boundary, the object was reflected on the boundary instead. The rotation of the object also followed a random walk, where the steps are drawn from δ φ ∼ N (0, (0.035 e ) 2 ).
Semantic representation network.
The semantic network consists of converging layers of SFA nodes. Information is first extracted locally and then integrated into more global and abstract features, see [ 55 ] for a more detailed description. In each SFA node, the same processing steps are performed ( Fig 1C , top right). The network was implemented using the MDP library [ 58 ]. It was trained sequentially from bottom to top on sequences of 10,000 images in each training session. Although SFA learns on sequences and the movement statistics determines which features are learned, SFA does not learn the movement statistics of the training sequences itself. In fact, the network learns to extract a semantic representation from a single input pattern, i.e., the extracted functions g ( i ) operate on single input patterns. This makes SFA fundamentally different from low-pass filtering.
Due to our particular choice of the object’s movement parameters in the training sequences (mainly the translation and rotation speeds), the four slowest features that emerged from the trained SFA network are related to the coordinates of the object’s center and its orientation [ 59 ]. To illustrate the SFA output, we used input sequences where the object moves along a trajectory and rotates by 360 degrees ( Fig 1D ). We refer to the vector y i , t of SFA features at a given time t in sequence i as the semantic, i.e., more abstract, representation of a single input image. After the semantic representation network had been trained, we used it to process sequences with different movement statistics. The temporal sequence of these semantic representation { y i ,1 , y i ,2 , y i ,3 , …}, describing the movement of the object in the input sequence i , is stored in the episodic memory system.
Sequence storage network.
Modeling the effect of adult neurogenesis in episodic memory storage
Top: Schematic of three stored sequences in the memory model, where the first elements in sequences 2 and i are similiar to each other. A: Without adult neurogenesis, the memory patterns are located in close proximity to each other in the memory space. B: In the asymptomatic state with a normal rate of adult neurogenesis, the augmentation with distinct pattern separation vectors distributes the sequences along an additional dimension in memory space. C: In the depressive state, the new sequence ( i ) is stored by re-using a pattern separation vector that had been assigned to a memory stored in a preceding asymptomatic state, based on the similarity of their first patterns. As a result, the two memory sequences, 2 and i , are more likely to interfere during retrieval.
https://doi.org/10.1371/journal.pone.0198406.g002
Modeling memory storage and retrieval in different disease states in MDD
In this study, we limit ourselves to considering only the asymptomatic state (A) and the depressive state (D). Based on the experimental evidence discussed above, we assume that the rate of AN is normal in the asymptomatic state and zero in the depressive state. The latter assumption implies that no new pattern separation vectors are generated for new sequences in the depressed state and previously generated ones are re-used. Four cases can be distinguished in principle based on which of the two states a memory sequence was stored and retrieved in ( Fig 3 ). We use the notation “X|Y” to indicate that a memory was stored in state X and retrieved in state Y. The four possible cases are A|A, A|D, D|D, and D|A. We will only discuss the first three cases, because the D|A case can be decomposed into those memories for which A|A applies and those for which D|D applies. We return to this issue in the Discussion.
The rate of adult neurogenesis (AN) is normal in the asymptomatic state and reduced in the depressive state. The origin of the arrow indicates during which state the memory was stored, and the termination of the arrow indicates during which state the memory is retrieved. A|A: memories stored and retrieved in the asymptomatic state; A|D: memories stored in the asymptomatic state and retrieved in the depressive state; D|D: memories stored and retrieved in the depressive state.
https://doi.org/10.1371/journal.pone.0198406.g003
Pattern separation improves the robustness of memory retrieval
We study the effect of augmenting memory patterns y i , t with a pattern separation vector a i on pattern separation in our model. Since the Euclidean distance between patterns plays an important role in retrieval in our model, we quantified the dissimilarity of patterns using the Euclidean distance. We find that distance between augmented patterns D a are larger than the distance between the original target patterns D t ( Fig 4 ), indicating that pattern separation indeed occurs in our model. Furthermore, the effect of pattern separation is largest for highly similar patterns ( D t < 1) and for large variability in the pattern separation vector (large σ a ).
Left: The distance between pairs of augmented patterns ( D a ), i.e., containing the pattern separation vectors, against the distance between pairs of original patterns ( D t ). A curve above the diagonal means that the augmented vectors and more dissimilar than the original vectors, indicative of pattern separation. Right: Same data as left panel, but plotted to emphasize pattern separation (= D a − D t ).
https://doi.org/10.1371/journal.pone.0198406.g004
A: Example performance of single-pattern retrieval across different level of retrieval noise (raw data). B: Distribution of the distance between cued and retrieved patterns at different levels of pattern separation σ a (only for the data within the dashed rectangle in A) as indicated by different colors. The legend is given in panel C, the reference σ a = 0 is shown in dark blue. C: Average performance of single-pattern retrieval as a function of retrieval noise. D: Retrieval error for retrieval of sequences at different levels of σ a (100 stored sequences, σ n = 0.1, a : 2-D).
https://doi.org/10.1371/journal.pone.0198406.g005
Next, we analyzed how pattern separation affects the retrieval of memory sequences in a model that stored 100 sequences (random walk trajectory), each with an unique pattern separation vector. Consistent with the result for single pattern retrieval, introducing pattern separation into the memory network (0 ≤ σ a ≤ 1), increases the retrieval accuracy of memory sequences ( Fig 5D ). However, large amounts of pattern separation ( σ a > 1) do not yield further improvement of the retrieval performance, indicating that pattern separation cannot fully eliminate the retrieval error in our model. In our model, DG AN is modelled by the generation of pattern separation vectors, which is parametrized by σ a . Better memory performance for ( σ a > 0) therefore means that AN improves episodic memory.
Dynamics of memory retrieval in asymptomatic and depressive state
To test our hypothesis that a reduction of AN in DG induces pattern separation impairment, which in turn impairs episodic memory in depression, we study the retrieval quality of memories stored and retrieved in the asymptomatic and depressive states, respectively. Two hundred sequences are stored in each state. Specifically, we compared retrieval performance in the three cases: A|A, A|D and D|D for different levels of retrieval noise σ n and pattern separation σ a . At low levels of pattern separation σ a = 0.1, retrieval performance is comparable in the three cases ( Fig 6A ). Increasing the level of pattern separation (from left to right in Fig 6A ), while keeping the level of retrieval noise fixed, improves the retrieval performance in all three cases, but the degree of improvement differs. When memories are stored and retrieved in the asymptomatic state (A|A), memory performance is better than if memories were retrieved in the depressive phase (A|D), or stored and retrieved in the depressive phase (D|D). This finding indicates that depression impairs memory performance. The higher the level of retrieval noise is, the more pattern separation is required to yield an advantage of A|A, or conversely an negative impact of depression. For example, for σ n = 0.05, a difference is already apparent for σ a ≥ 0.1, while for σ n = 0.2, a difference is only apparent for σ a ≥ 0.5. If retrieval noise dominates, i.e., σ n ≥ 0.5, no amount of pattern separation yields a difference between A|A, A|D and D|D. We discuss these effects in more detail below. To rule out the probability that our results are specific to a particular input stimulus, we also studied the model with different objects (‘T’, ‘U’, ‘E’) as input stimuli and find very similar results (data not shown). Together, these results suggest that retrieval performance in our model depends on the mutual interaction between the retrieval noise and pattern separation and that a memory deficit in depression would not be expected in every case.
A: Retrieval performance for the three cases A|A, A|D, D|D (indicated by color) at different levels of pattern separation σ a (left to right) and retrieval noise σ n (top to bottom). B: The duration of depressive episode affects the retrieval performance of A|D and D|D. Duration is measured by the fraction of memories stored in the depressive episode k ( Eq 12 ). C: Increasing the number of stored sequences negatively impacts the retrieval performance in all cases, while the difference are preserved. D: Increasing the dimensionality of the pattern separation vector, up to a certain point, increases the difference between the A|A and the other cases. Values in B, C and D are calculated based on the 30 th element in the sequence ( σ a = 1, σ n = 0.1). For A,B,C: a :2-D; for A,C,D: k = 0.9; for A,B,D: 200 stored sequences in both asymptomatic and depressive state respectively.
https://doi.org/10.1371/journal.pone.0198406.g006
Impact of depressive episode duration on retrieval performance.
The results in Fig 6A were obtained with k = 0.9. Across all values of k , we found that the retrieval performance in the case of A|A is the most accurate, while D|D is the worst. The difference between the two cases becomes more prominent for larger k ( Fig 6B ). We also find that for short duration of depression (small k ), the retrieval performance of A|D is as good as the performance of A|A and then converges to the same level as D|D as the duration of the depressive episode increases (larger k ). This indicates that even the remote memories formed in earlier asymptomatic state of the depressive patients are impaired as depression lingers.
The role of other model parameters.
We studied the influence of two other parameters that have a potentially important role in memory performance in our model. First, we studied the role of the memory load by storing larger numbers of sequences in the memory network. Retrieval performance for all three cases becomes worse for higher memory load. The difference, however, between depressive state and asymptomatic state is almost constant ( Fig 6C ). Second, we expected the dimensionality of the pattern separation vector to influence pattern separation, i.e., higher dimensionality leads to larger pattern separation effects. Indeed, our results show that the advantage of the A|A case is already apparent with only a one dimensional pattern separation vector ( Fig 6D ). The effect is stronger for larger numbers of dimensions. However, for this particular set of memory sequences, increasing the dimensionality beyond two has little effect on memory performance in each of the three cases.
Accounting for the pattern of retrieval errors.
Next, we explored how the difference in retrieval accuracy among the three cases arises. A retrieval error occurs when the retrieved pattern is different from the stored one, in other words, when retrieval jumps to an incorrect pattern. Intuitively, one might expect that the more frequently incorrect jumps occur, the larger the retrieval error is, but we found previously that the retrieval error is dominated by another process, namely the sequence divergence [ 55 ]. It refers to the tendency of two sequences that are close to each other at some point in time to diverge from each other over time. Since memory patterns are retrieved sequentially in our model, the movement along the sequence exacerbates the retrieval error, if the incorrect sequence diverges from the correct one. We therefore examined the sequence divergence as well as the probability of jumps to an incorrect pattern within the same sequences ( p w ) and between two sequences ( p b ) during retrieval. Sequence divergence is quantified by the increase in the distance between the subsequent elements of two sequences after two patterns in the respective sequences were the closest patterns to each other [ 55 ].
Three observations account for the differences in retrieval error seen in Fig 6 . First, increasing the retrieval noise leads to more faulty transitions both within and between sequences ( Fig 7A , from top to bottom), which accounts for the increase in the retrieval error with increasing retrieval noise. Second, with the same level of retrieval noise, increasing the level of pattern separation ( σ a ) reduces the rate of faulty transitions between sequences, but increases the faulty transition rate within sequences. This is expected since pattern separation in our model acts to make sequences more distinct from each other. As a result, incorrect patterns within the same sequence are more often the closest element to the retrieval cue for the next element. This effect is more apparent in the A|A case than in the other two cases due to the stronger effect of pattern separation. Since jumps between sequences lead to larger errors than jumps within sequences, the differences in p b between the three cases account for the differences in the respective retrieval errors ( Fig 6A ), except for the lack of a difference at high levels of retrieval noise ( σ n = 0.5).
A:left, probability of incorrect jumps between sequences ( p b ); right, probability of incorrect jumps within sequences ( p w ). B: Sequence divergence. For A, B: a :2-D, k = 0.2, 200 stored sequences.
https://doi.org/10.1371/journal.pone.0198406.g007
The third observation fills this explanatory gap. Sequence divergence is maintained across different levels of pattern separation in the A|A case, while sequence divergence drops in the other two cases ( Fig 7B ). The latter effect is the result of reusing pattern separation vectors based on the similarity of the sequences in the A|D and D|D cases. Through this mechanism similar sequences become more clustered. Since pattern separation drives these clusters further apart, incorrect jumps go to similar sequences, thus reducing sequence divergence, when pattern separation is high. The lower sequence divergence offsets the higher jump probability p b in the A|D and D|D cases and therefore reduces the difference to the A|A case in the retrieval error, but only if the jump probability p b for the A|A case is not already close to zero. These conditions are satisfied for all levels of pattern separation, when σ n = 0.5, which explains why the A|A case performs no better in this noise regime.
Pattern separation at input stage
After showing that pattern separation improves episodic retrieval in our model, we asked whether pattern separation has to occur in the memory system or whether it could instead occur in the sensory system before the patterns are processed by the memory system. To study this question, we randomly flipped different numbers of pixels of each input image in the testing data ( Fig 8A ). No noise was added to the memory representations during storage. The way we added noise to the input patterns followed the same strategy that we used for pattern separation in the previous simulations. That is, the same pixels are flipped for all patterns within the same sequences, whereas different sets of pixels are flipped for the patterns in different sequences. Therefore, similar input patterns in different sequences should be separated. We tested whether this kind of pattern separation alleviates the interference between memories and facilitates the accuracy of memory retrieval.
A: Example of the manipulated input patterns. Top: same pattern as in Fig 1B , but with 5% pixels flipped (300 × 300 pixels); Bottom: the scaled version. B: Retrieval error as a function of the fraction of randomly flipped pixels in the input image ( σ n = 0.2, 200 stored sequences). Dashed curve: retrieval performance of the model with neurogenesis ( σ a = 1) for comparison. C: With the same amount of noise in the input (1% flipped pixels), retrieval error increases monotonically with increasing retrieval noise σ n . D: The difference between the retrieval error for original patterns and that for noisy input pattern gradually increases with input noise ( σ n = 0.2). Values in C and D are drawn from the 30 th element in the sequence.
https://doi.org/10.1371/journal.pone.0198406.g008
Unlike what one might expect, we found that the retrieval performance is impaired by pattern separation in the sensory inputs ( Fig 8B and 8D ). Similar to previous results, retrieval noise impairs the retrieval performance ( Fig 8C ). These results indicate that pattern separation of sensory inputs does not necessarily mean the semantic representation patterns are separated as well, since the SFA network is performing a nonlinear operation. These results are consistent with our previous study [ 55 ], where we found that the episodic retrieval is more accurate when the semantic network is trained on the same image statistics that generates the inputs to be stored in memory, as compared to when the image statistics differ. Episodic retrieval is impaired when we add noise to the input, because by doing so we changed the input statistics after the semantic network had been trained. We therefore conclude that pattern separation at the sensory stage is not effective and therefore has to occur in the memory system.
We have developed a computational model to study episodic memory deficits in MDD. We assumed that MDD is associated with a reduction of AN in the DG, and that this reduction in AN impairs pattern separation. We hypothesized that the impairment of pattern separation in turn reduces the accuracy of episodic memory retrieval. In our model, episodic memories are encoded based on a semantic representation of the sensory inputs [ 55 ]. We investigated episodic memory deficit in MDD with an intact semantic system, which is consistent with observations that semantic memory is not affected in MDD [ 11 , 12 ]. Our model of episodic memory is built around the idea that episodic memories are best represented by sequences of neural activity patterns [ 28 , 29 , 56 , 63 ]. This aspect distinguishes our model from other models of neurogenesis, which only consider the storage and retrieval of static patterns.
Correspondence to neuronal mechanisms underlying pattern separation
Even though our model does not reflect the anatomy and physiology of the hippocampus, it nevertheless describes hippocampal function at an abstract level and the functions of our abstract model can be roughly mapped onto the hippocamal circuit. The hippocampus has been found to be essential for sequence memory [ 64 , 65 ] and we previously hypothesized that the hippocampal circuit is optimized for storing sequences of neural activity patterns [ 28 ]. During episodic memory storage, input patterns are mapped onto pre-existing intrinsic sequences of neural activity in CA3. In CA3, sequences are thought to be generated by the dynamics of its recurrent network, e.g. [ 66 ]. A given state of the network drives the next state through the recurrent synapses. In our model, we approximate this process in our sequence retrieval network, where the sequence elements are linked by associating each element with a retrieval key for the next element. Thus, the sequence elements y i , t correspond to activity patterns in CA3.
In our model, the pattern separation vector is identical for all patterns within the same sequence, while different pattern separation vectors are generated for different sequences. This assumption is consistent with the temporal tagging hypothesis [ 51 ]. It was proposed that memories formed at distinct times would be represented by different groups of neurons in DG since newborn cells continue to be integrated into the network. As a result, memories formed close in time would be associated by the same group of immature DG granule cells (pattern integration), while memories formed at times far apart would be represented by distinct sets of DG neurons. Similarly, the functional cluster hypothesis proposes that the same contexts are represented by DG cells that were born simultaneously [ 43 ]. We therefore conclude that our abstract model is firmly rooted in the neuronal mechanisms underlying pattern separation in the hippocampal formation.
Rate of adult neurogenesis and memory persistence
Empirical evidence suggests that increases in the rate of AN improves the performance on a variety of memory tasks [ 24 , 41 ]. Here, we find that increasing AN up to a certain level improves memory performance (Figs 5 and 6 ). Moreover, since retrieval performance in our model depends on the interaction between the retrieval noise and pattern separation, memory deficits would not be expected in every case of MDD. Indeed, some studies failed to find episodic memory deficits in depressed individuals [ 72 , 73 ]. We hypothesize that the retrieval error in our model is determined by task demands, the subject’s level of engagement, and neural processing. Pattern separation would be affected by the rate of DG AN, and the severity, and perhaps the duration, of the depressive phase. To test these predictions, future experimental studies could systematically vary the rate of AN and retrieval noise, and measure the affect of these manipulations on retrieval performance.
What is currently missing from our model is a detrimental effect of AN on memory. Experimental [ 47 , 74 ] and computational [ 47 , 75 ] studies have found that a high rate of AN leads to faster forgetting. Apparently, integrating new neurons into the hippocampal circuit affects memories that are already stored, because new cells and new connections compete with existing ones. In other words, there is trade-off between plasticity and stability.
Episodic memory deficits in MDD
Our model predicts that MDD has an retrograde effect on episodic memory retrieval ( Fig 6B ). That is, memories retrieved in a depressive state are less accurate, even if they had been stored in a preceding asymptomatic state (A|D), as compared to memories that were stored and retrieved in an asymptomatic state (A|A). Studies of auto-biographical memories, which we discuss below, appear to support a retrograde effect of MDD on previously formed memories. However, to the best of our knowledge, a retrograde effect has yet to be demonstrated under laboratory-controlled conditions. Moreover, we find that memory deficits depend on the duration of the depressive episode. The longer the depressive episode lasts, the more severe the memory performance becomes.
In addition to the three case discussed in our study (A|A, A|D, D|D), there is another possible scenario. A memory can be stored in the depressive state and retrieved in the asymptomatic state (D|A). While this case is distinct from the other three, we did not include it in our study because it can be viewed as a composite of two other cases. Memories stored in the depressive state are not assigned a distinct pattern separation vector, while memories stored in the subsequent asymptomatic state are. New memories would therefore rarely interfere with previously stored memories and the D|A case can be decomposed into those memories that fall under the A|A case (new memories) and those under the D|D case (old memories). Our model, therefore, predicts that the memory deficit is not rescued when the depressive state ends. In other words, the damage caused in the depressive state by interference in the memory system cannot be undone. By contrast, the A|D case cannot be decomposed, because the pattern separation vector generated during the asymptomatic phase are re-used during the depressive phase, which leads to retrograde interference.
We found that the type of error committed during memory retrieval differs during MDD ( Fig 7 ). According to our model during MDD patients might more frequently confuse memories formed at different timepoints than healthy controls. Somewhat paradoxically, it also predicts that controls incorrectly report events that occurred close in time more frequently than patients do. This novel prediction awaits testing in experimental studies.
Shifting from episodic to semantic memory in MDD
Apart from impairments in episodic memory, patients suffering from MDD also show over-general memories [ 12 , 76 – 78 ]. When subjects are asked to recall a particular event from their personal history related to a given cue, patients, more often than controls, retrieve rather general information that summarizes a category of events [ 12 , 77 ]. This is called the over-general memory effect. For instance, when cued with “enjoy” to recall an event, patients tend to produce generic answers, e.g., “I enjoy a good party”, whereas controls produce specific memories such as “I enjoyed Jane’s party last Saturday”. To account for this effect, Williams et al. [ 77 ] adopted the Conway and Pleydell-Pearce model [ 79 ], which suggests that autobiographical memories are arranged in a hierarchical structure with the general categories at the top, specific categories in the middle and specific event memories at the bottom. Autobiographical memories are retrieved by traversing this memory structure from top-to-bottom. Williams et al. suggest that MDD patients block the access to specific event memories in order to avoid retrieving painful memories and therefore end the retrieval process at an abstract level.
By contrast, we propose that the same episodic memory deficit that we studied here might be sufficient to account for over-general memories, too. Episodic memories together with personal semantic information forms autobiographical memory. Episodic memories are about specific events, whereas semantic memories refer to general facts. Therefore, over-general memory can be seen as a shift from the retrieval of episodic memories to the retrieval of semantic memories. If episodic memory retrieval is impaired during MDD, retrieval of autobiographical memories is more likely to result in a semantic memory which is mostly preserved during MDD. This shift from a reliance on episoidic memory to reliance on semantic memory appears as a shift from specific to over-general memories. This account is consistent with a previous suggestion that over-general memory could result from reduced episodic recall, increased semantic recall or the combination of both [ 12 ].
In conclusion, the model we present here might be able to account for both over-general memories and episodic memory deficits in MDD.
Supporting information
S1 file. python code..
https://doi.org/10.1371/journal.pone.0198406.s001
Acknowledgments
We thank Sonakchhi Shrestha for support in performing the computer simulations. This work was supported by grants from the Stiftung Mercator, from the German Research Foundation (DFG) through the SFB 874, project B2, and from the German Federal Ministry of Education and Research (BMBF), grant 01GQ1506.
- View Article
- PubMed/NCBI
- Google Scholar
- 5. Burt DB, Zembar MJ, Niederehe G. Depression and memory impairment: a meta-analysis of the association, its pattern, and specificity. Psychological Bulletin. 1995;.
- 27. Amaral D, Lavenex P. Hippocampal Neuroanatomy. In: The Hippocampus Book. Oxford University Press; 2006. p. 37–114.
- 54. Cheng S. Consolidation of Episodic Memory: An Epiphenomenon of Semantic Learning. In: Axmacher N, Rasch B, editors. Cognitive Neuroscience of Memory Consolidation. Cham, Switzerland: Springer International Publishing; 2017. p. 57–72.
- 76. Conway M, Williams H. Autobiographical memory. In: Learning and Memory: A Comprehensive Reference. Oxford:Elsevier Ltd; 2008. p. 893–909.
The Future of Depression Treatment: The Neurogenesis Theory

Some studies show that antidepressants act by triggering neurogenesis. Source: http://en.wikipedia.org/wiki/File:GFPneuron.png
Nearly half of all clinically depressed patients fail to respond to available antidepressant medications (1). Though antidepressants are effective for some depressed patients, this selective efficacy is still not fully understood. Professor Poul Videbech, a specialist at the Centre for Psychiatric Research at Aarhus University Hospital, has dedicated himself to researching the effects of depression to better understand the mechanism of antidepressants. (2)
In one project, Videbech scanned the brains of depressed patients to observe structural effects of the disorder. Videbech concluded, “My review shows that a depression leaves its mark on the brain as it results in a ten percent reduction of the hippocampus… In some cases this reduction continues when the depression itself is over” (2). Videbech believes that nerve reduction supports the neurogenesis theory of depression which posits that depression results in a cessation of neuron birth in the brain (3). Support for this theory lies in the fact that, with extended use, antidepressants trigger neurogenesis by initiating the birth of new nerve cells. Studies at the Centre for Psychiatric Research, where patients suffering from depression were followed for ten years using brain scans, demonstrate that shrinking of the hippocampus is reversible when depressed patients are treated.
People not suffering from depression have a balance in degradation and regeneration processes in the brain. The degradation process refers to the breaking down of nerve cells, while regeneration refers to the formation of nerve cells (1). Depressed patients show greater activity in the degradation system, which explains Videbech’s findings that brain structures are reduced in patients with depression. The location of reduction cited by Videbech is the hippocampus, the structure of the brain responsible for the storage and retrieval of memories. Hippocampal reduction explains the common symptom of memory problems in patients with depression. With antidepressant use, and hence a return of neurogenesis, memory problems and depressive symptoms are reduced. Meaning, boosting neurogenesis results in a returned balance between the degradation and regeneration processes. (2)
The most common form of antidepressants, serotonin reuptake inhibitors (SSRIs), were believed to have their effect by boosting levels of serotonin in the brain. However, scientists have proven that SSRIs take about a month to improve mood in depressed patients. This delay in treatment suggests that another process, one influenced by serotonin, is involved. The neurogenesis theory of depression explains that the delay in mood improvement is a result of the minimal effect serotonin has on neurogenesis. Researchers have turned their focus to chemicals in the brain that promote neurogenesis and suggest that new treatments targeting said chemicals could be a more logical and effective treatment for depression. (1)
The neurogenesis theory has been supported with animal studies. In one trial, researchers induced a depression-like condition in mice so they develop a depressive behavioral pattern. The mice were then given antidepressants and normal behavior returned. Then, when the mice were subjected to radiation treatment, a process known to terminate the formation of new nerve cells, the antidepressants stopped working and the mice returned to their depressed behaviors (1). Videbech cites this study as proof that antidepressants are only effective because of their influence on neurogenesis and when that influence is eliminated, antidepressants no longer improve depressive symptoms (2).
A recent article in Nature Medicine cites a promising new line of research in the ceramide system. In one study, after mice took Prozac-like antidepressants the levels of ceramide, a fat molecule in the brain, significantly decreased. In the brain, ceramide blocks brain cell growth. Meaning, Prozac affected ceramide levels which in turn increased neurogenesis. Scientists believe that further research on molecules like ceramide will continue, and eventually result in, more direct and effective antidepressant treatments. (4)
References:
1. A. Maxmen, Psychol. Today. 64, 39-40 (2013).
2. S. Hildebrandt, Depression Can Damage the Brain (2011). Available at http://sciencenordic.com/depression-can-damage-brain (19 December 2013).
3. I. Dell, Depression: Neurogenesis and Depression (2010). Available at http://sites.lafayette.edu/neur401-sp10/applications-in-health-and-medicine/depression/ (19 December 2013).
4. E. Gulbins et al, Nat. Med. 19, 934-938 (2013).
Leave a Reply Cancel reply
Your email address will not be published. Required fields are marked *
Save my name, email, and website in this browser for the next time I comment.
- Bovine Brain Damage: New Findings in the Field of Wildlife Neuroscience
- The Heredity of Mental Disorders
- Levels of Empathy in Apes and Humans
- Stochastic Volatility Models and its Effect on the Asset Market
- Corporate Psychopathy: Does Empathy Cripple Leaders?
- Dementia Villages – Experimenting with Universal Design Treatment

An official website of the United States government
The .gov means it’s official. Federal government websites often end in .gov or .mil. Before sharing sensitive information, make sure you’re on a federal government site.
The site is secure. The https:// ensures that you are connecting to the official website and that any information you provide is encrypted and transmitted securely.
- Publications
- Account settings
Preview improvements coming to the PMC website in October 2024. Learn More or Try it out now .
- Advanced Search
- Journal List
- HHS Author Manuscripts

Neuroplasticity in cognitive and psychological mechanisms of depression: An integrative model
Rebecca b. price.
1 Departments of Psychiatry and Psychology, University of Pittsburgh, Pittsburgh, PA, USA
Ronald Duman
2 Department of Psychiatry, Yale University, New Haven, CT
Chronic stress and depressive-like behaviors in basic neuroscience research have been associated with impairments of neuroplasticity, such as neuronal atrophy and synaptic loss in the medial prefrontal cortex (mPFC) and hippocampus. The current review presents a novel integrative model of neuroplasticity as a multi-domain neurobiological, cognitive, and psychological construct relevant in depression and other related disorders of negative affect (e.g., anxiety). We delineate a working conceptual model in which synaptic plasticity deficits described in animal models are integrated and conceptually linked with human patient findings from cognitive science and clinical psychology. We review relevant reports including neuroimaging findings (e.g., decreased functional connectivity in prefrontal-limbic circuits), cognitive deficits (e.g., executive function and memory impairments), affective information processing patterns (e.g., rigid, negative biases in attention, memory, interpretations, and self-associations), and patient-reported symptoms (perseverative, inflexible thought patterns; inflexible and maladaptive behaviors). Finally, we incorporate discussion of integrative research methods capable of building additional direct empirical support, including using rapid-acting treatments (e.g., ketamine) as a means to test this integrative model by attempting to simultaneously reverse these deficits across levels of analysis.
Introduction
Depression is the leading cause of disability worldwide with a public disease burden of staggering proportions 1 . While efficacious treatments have been available for decades, remission rates are low, relapse rates are high, and disorder prevalence rates remain notably stagnant, with only 12.7% of patients receiving minimally adequate treatment 2 . At the molecular level, depression has been characterized as a failure of neuroplasticity, including neuronal atrophy and synaptic depression in the medial prefrontal cortex (mPFC) and hippocampus 3 – 5 . At the neurocognitive level, depression has been called a disorder of impaired cognitive flexibility and prefrontal inhibition 6 – 8 , leading to inflexible negative biases in cognition, such as rigidly held negative beliefs 9 .
Impaired neuroplasticity is theorized to underlie depression, but an empirical divide separates molecular models from cognitive/information processing models that motivate gold-standard behavioral treatments for depression. In this integrative review, we propose a model of neuroplasticity as a multi-level construct, conceptually linking relevant empirical findings across molecular/neuronal, neural network, cognitive, implicit information processing, and clinical levels of analysis. We highlight research approaches that help to bridge this divide. As an example, we discuss the potential for ketamine—which exhibits both rapid plasticity-enhancing effects in animal models 4 , 10 and rapid clinical effects in human patients 11 , 12 —to provide a test of the predictions of this integrative model, including simultaneous and correlated reversals of multiple plasticity-related deficits across levels of analysis.
Neuroplasticity models of depression
Studies of the molecular and cellular mechanisms underlying depressive-like behaviors in rodent models and convergent brain imaging and postmortem studies of depressed patients have provided significant advances in our understanding of mood disorders. These findings reveal alterations at the levels of intracellular signaling, gene expression, neurotrophic factors, neurogenesis, neuroinflammation, excitatory and inhibitory neurotransmission, and synaptic number and function, and have been described in several brain regions implicated in depression 13 – 22 . The signaling pathways and types of molecular and cellular events vary depending on the brain regions studied. Studies have focused on PFC, hippocampus, amygdala, the ventral tegmental area-nucleus accumbens (VTA-NAc) dopamine system, and the HPA axis. These findings have resulted in complementary theories of depression and antidepressant response that have been linked, either directly or indirectly, to the molecular and cellular signaling mechanisms that mediate synaptic plasticity, and have therefore contributed to a broader neuroplasticity hypothesis of depression 3 – 5 . One of the leading theories highlights the roles of the PFC and hippocampus, including disruption of neurotrophic factors and synaptic connectivity that are related to neuroplasticity mechanisms 4 , 5 . According to this model of depression, chronic stress leads to sustained decreases in neuroprotective factors [e.g., brain-derived neurotrophic factor (BDNF) expression and signaling] that damage or hinder plasticity, fostering neuronal atrophy and decreased synaptic number and function, particularly in the mPFC and hippocampus 3 , 4 . This results in deficient adaptation to the environment, compromising learning and stress coping, and to downstream gain of activity in some ‘limbic network’ regions regulated by the PFC. One of the key efferent targets of mPFC is the amygdala, a region involved in control of fear and anxiety and widely implicated in human depression 23 ; other output regions include the dorsal raphe, which has been linked to helplessness behavioral deficits (e.g., loss of control); the lateral habenula, associated with anhedonic and aversive responses; and the bed nucleus of the stria terminalis, another region linked with anxiety and negative emotion 22 . Conversely, when neuroplasticity is enhanced (e.g., by treatment), synaptic contacts increase, enhancing adaptability by allowing activity-dependent competition to stabilize the neural structures that best represent internal and external conditions 24 – 26 . These basic neuroscience findings are linked directly to shifts in depression-like behaviors in animal models, such as performance on the forced swim test, a probe of “despair,” the novelty suppressed feed test, a probe of “anxiety”, and the sucrose preference test, a probe of “anhedonia.” 27
Molecular and cellular studies have examined the intracellular signaling pathways underlying the regulation of synaptic function by stress and antidepressant treatments. Repeated stress decreases the expression of BDNF in limbic and cortical brain regions, notably the hippocampus and PFC 4 , 5 , 22 . In addition, repeated stress exposure decreases mTORC1 signaling, which is required for synapse formation and neuroplasticity 28 , and inhibition of mTORC1 decreases synapse formation in the PFC and is sufficient to cause depression-like behaviors in rodents in the absence of stress exposure, demonstrating a causal relationship between synapses and behavior. Recent evidence demonstrates that chronic stress also leads to activation of microglia, the brain’s resident immune cells, which engulf synapses on nearby pyramidal neurons and thereby contribute to neuronal atrophy 29 . It is also notable that in some brain circuits, stress and depression may lead to enhancement of neuroplasticity mechanisms. For example, studies demonstrate that social defeat stress increases BDNF in the VTA-NAc pathway, leading to enhanced function that is thought to contribute to disruption of reward and motivation behaviors in depression 20 , 21 . There is also evidence that repeated stress causes hypertrophy of pyramidal neurons in the basolateral nucleus of the amygdala that could contribute to altered anxiety and emotion 30 , 31 . These findings demonstrate the diversity of the disruptions of plasticity in depression that vary according to brain circuitry and the underlying function regulated by different brain regions.
Clinical evidence provides some further support for the relevance of neuroplasticity mechanisms in depressed patients, though not all findings are consistent. Ketamine, a glutamatergic agent used routinely for induction and maintenance of anesthesia, exhibits well-replicated, rapid, potent antidepressant effects in randomized controlled trials (i.e., metaanalytic Cohen’s d =1.4, a large effect) 12 , even in difficult-to-treat conditions such as treatment-resistant depression 32 and bipolar depression 33 . In addition, the FDA has recently approved a nasal application of (S)-ketamine, referred to as esketamine (Spravato), for treatment resistant depression. The rapidity and magnitude of ketamine’s effects have been attributed to its ability to rapidly reverse neuroplasticity deficits in animal models 3 , 4 , 10 , 34 . A single dose of ketamine increases BDNF release and stimulates mTORC1 signaling, which leads to increased levels of synaptic proteins (i.e., GluR1, PSD95, and synapsin 1) and increased number and function of synapses in the PFC 4 , 22 , 35 . An elegant recent study provided further evidence that new synapse formation is causally related to the antidepressant actions of ketamine 10 . Using in vivo two photon imaging, ketamine reversed the loss of synapses caused by stress, while selective deletion of these new synapses blocked the sustained antidepressant-like behavioral actions of ketamine.
Of note, however, rapastinel, another drug that reverses synaptic plasticity deficits and exhibits antidepressant-like effects in rodent models 36 , 37 , has been studied in 3 clinical trials to date with relatively weak evidence for its efficacy 38 . Given that clinical studies in depression are subject to well-known confounds including strong placebo responsivity and heterogeneous clinical presentations, further trials may be warranted to clarify rapastinel’s antidepressant efficacy. Regarding the specific role of mTORC1, a recent preliminary study 39 found that rapamycin, an agent capable of blocking mTORC1, when given concurrent to ketamine infusion, did not block ketamine’s antidepressant efficacy (as was expected), but rather extended the window of ketamine’s antidepressant effect. However, the authors speculated that the dose of rapamycin (6 mg oral) may in fact have been insufficient to block mTORC1 in the brain, and that rapamycin’s potent, peripheral anti-inflammatory actions most likely account for the observed paradoxical effect. Both basic and clinical research have vital and complementary roles to play in the ongoing developing, testing, and refining of neuroplasticity theories of depression.
Integrative hypothesis
In spite of these findings suggesting crucial links between neuroplasticity mechanisms and behavioral tests in animal models, a fundamental translational question remains with respect to the alleviation of complex, multifaceted human conditions: how, precisely, might neuroplasticity mechanisms profoundly alter human experience? Our focus in the current review is to specify potential downstream results of molecular plasticity impairment observable at more ‘macro’ levels of analysis. In humans, depression and chronic negative affect are associated not only with decreases in convergent molecular and cellular neuroplasticity markers (e.g., BDNF 40 ; prefrontal synapses measured post-mortem 41 ), but also with altered functional integration across PFC and limbic (e.g., hippocampus, amygdala, striatum) circuits 8 , 42 – 44 . Such neural network alterations are posited to contribute to impaired regulatory control of stimulus-driven affective processing 7 , 45 , producing rigid negative biases evident across a wide range of implicit information processing domains (e.g., negative appraisals of self, the environment, and the future 46 ; preferential attention and memory for negative stimuli 45 , 47 ). These neural and implicit cognitive patterns may in turn contribute to impaired overall cognitive and behavioral flexibility 6 , 48 and help to maintain and reinforce a state of high negative affect by fostering overestimation of the personal shortcomings, dangers, and misfortunes inherent to the individual’s life 9 . Although psychological and neurocognitive accounts of depression have not typically been united with neuroplasticity findings into a single integrative theory, strong parallels are suggested by overlap in the implicated brain regions (e.g., mPFC, hippocampus, amygdala) and behavioral sequelae (rigid responses to the environment). On this basis, we propose an integrative model of neuroplasticity and mood that bridges these levels of analysis ( Table 1 ; Figure 1 ).

Regions with prominent neuroplasticity deficits in animal models of depression 4 , 5 (in green) and functionally interconnected regions within a cortico-mesolimbic circuit relevant to mood regulation (blue). Some proposed functions of these regions with relevance in our integrative model are highlighted. “Medial prefrontal cortex (PFC),” as implicated in animal models, includes a number of subdivisions implicated in human depression including subgenual anterior cingulate cortex (ACC) and ventro- and dorso-medial PFC areas. Dashed lines represent primary hypothesized impairments in prefrontal cortex connectivity and top-down regulation of limbic regions, resulting in impairments in behavioral and cognitive flexibility across levels of analysis.
Neuroplasticity Markers Across Levels of Analysis
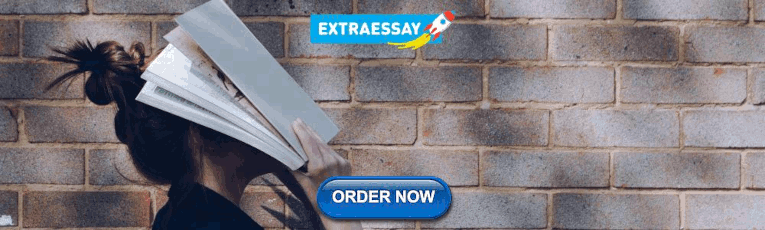
Plasticity impairments in neural networks
Consistent with the predictions of animal models, hippocampal and PFC volumes are robustly decreased in depressed patients, according to in vivo structural imaging 5 . Recently compiled large imaging corpuses, which include >1000 unipolar depressed patients and many thousands of healthy control participants, have documented particularly robust decreases in hippocampal volume 49 , which were driven by individuals with recurrent depression and early age of onset (≤age 21), suggesting the impact of the depressed state on hippocampal volume may be cumulative across time and/or episodes. Amygdalar volumes were likewise decreased, but only in patients with early-onset depression, and this finding was not statistically robust after correcting for multiple comparisons. In PFC subregions, robust decreases in cortical thickness were observed in medial and orbital areas of the PFC and anterior cingulate cortex (ACC) 50 , which constitute subdivisions of the rodent “mPFC” homologue ( Figure 1 ). Convergent meta-analytic findings suggest disrupted white matter microarchitecture in depressed patients in key white matter tracts that facilitate inter- and intra-hemispheric integration across PFC and limbic regions, including the corpus collosum, front-occipital fasciculus, and PFC projection fibers 51 .
Given that brain structure constrains functional networks 52 , these volumetric changes are hypothesized to underlie abnormalities observed in functional neuroimaging measures within the same circuits. Brain processes may best be characterized as the coordinated activity of disparate brain regions over time 53 . Functional connectivity measures (i.e., the temporal correlation between spatially remote neurophysiological events) may be a particularly relevant marker of plasticity deficits, as these indices directly quantify the degree to which regions of the brain act in a coherent fashion and/or influence one another over a second-to-second timeframe. Thus, connectivity indices, often measured with neuroimaging methods such as fMRI, may provide a crucial intermediary, helping to bridge structural neuroplasticity markers (e.g., synaptic dysconnectivity) to downstream alterations in the brain’s ‘output,’ i.e., mental and cognitive processes. When using meta-analytic techniques to identify the most robust patterns across individual studies, depression has been associated with decreased ‘intrinsic’ functional connectivity (i.e., connectivity observed during a resting state) within and between PFC and limbic networks, including decreased connectivity between mPFC and limbic/affective regions including the hippocampus and amygdala 54 . Depression has likewise been linked to decreased ‘global’ intrinsic connectivity between the medial and lateral PFC and all other regions of the brain 55 , and to decreased inter- and intra-hemispheric integration within and across dorsolateral PFC, medial PFC/ACC, hippocampus, and parietal regions 56 , 57 . Notably, these connectivity decreases are evident in spite of simultaneously increased intrinsic connectivity in other, spatially overlapping networks (e.g., within the midline “default mode network” 54 , 58 ), suggesting connectivity aberrations in depression are circuit-specific.
Similarly, during task states requiring processing of affective stimuli (e.g., evaluating the personal relevance of negative/positive attributes; viewing negative pictures), depressed patients have shown decreased functional connectivity between lateral PFC and amygdala 44 and/or hippocampus 59 and across medial PFC/ACC and limbic regions 60 , 61 . During ‘cognitive reappraisal’ tasks, in which volitional attempts are made to down-regulate subjective negative responses to negative stimuli 62 , depressed patients have shown decreased activation in lateral and medial PFC coupled with increases in amygdalar response 63 , 64 , and altered mPFC-amygdalar connectivity 65 , suggesting impaired volitional PFC regulation of the amygdala. Collectively, these patterns observed in human patients are consistent with the premise that neuronal and molecular plasticity deficits may feed forward to impairments in the coordinated function of prefrontal and limbic regions 66 , resulting in impaired PFC regulation and unchecked stimulus-driven responses to salient negative stimuli.
Plasticity impairments in cognition
Structural and neural circuit alterations described above may contribute to performance deficits observed in depressed patients during cognitive and neuropsychological tasks. These deficits are consistent with an impaired capacity to engage flexibly with external stimuli and efficiently exert goal-directed cognition in support of task demands, thereby reducing flexible, adaptive behavior in complex environments 67 . Meta-analysis of executive functions in depressed patients suggest pronounced deficits (Cohen’s d ≥1.0) in cognitive flexibility, inhibition capacity, and verbal fluency, as well as moderate impairments in strategic planning and organization 68 , 69 . Attention and concentration are also broadly impaired 70 , 71 . These wide-ranging executive deficits suggest a broad impairment in the ability to control and regulate other lower-order functions and behaviors, including the ability to initiate and stop actions, to monitor and change behavior to match shifting demands of the environment, and to plan optimal behaviors in the face of novelty 69 . Such abilities rely on the integrity and coordinated function of both lateral and medial PFC and ACC subregions 72 , 73 , paralleling the neuronal and neural network levels (as described above), and are believed to contribute fundamentally to depressed patients’ substantial functional impairments 74 . Correspondingly, computerized cognitive training interventions, designed to rehabilitate core prefrontal cognitive functions (e.g., working memory) through repeated task practice, show preliminary meta-analytic evidence of acute efficacy in the treatment of depression, including moderate effects on symptom severity and daily functioning 75 , 76 .
Memory deficits are also apparent in depressed patients. These include impairments in both prospective memory for new information—across a variety of domains and task conditions 77 —and retrospective autobiographical memory retrieval, wherein a lack of specificity in retrieved memories (“overgeneral memory”) has been described 78 . Overgeneral memory is correlated with reduced problem-solving performance 79 and imageability of future events 80 , suggesting the lack of specificity in recalling past life events constrains the ‘cognitive set’ of viable possible actions that are readily available to conscious awareness. Memory deficits in depressed patients have long been hypothesized to relate directly to neuroplasticity deficits within the hippocampus 81 , given its key role in memory formation and retrieval. However, circuit-level dysfunctions impacting connectivity within and across multiple PFC and limbic regions may best explain the breadth of cognitive impairments observed in depression, collectively reducing the individual’s capacity to flexibly adjust in response to a continually changing environment.
Plasticity impairments in affective information processing patterns
In addition to cognitive deficits on neuropsychological tests (utilizing standardized, ostensibly neutral stimuli), depression is characterized by rigid, valence-specific biases in the processing of affective information. These biases create preferential processing of negative information (i.e., up-regulation of the ‘negative valence system’), and decreased engagement with positive information (down-regulation of the ‘positive valence system’), across a range of information processing domains, including attention, memory, interpretation, implicit associations (e.g., negative representations of one’s self), and learning and decision-making 8 , 82 .
Attention, which acts as an initial filter on the information entering conscious awareness, shows valence-specific alterations in depressed patients including attentional preference for dysphoric stimuli and biases away from positive stimuli 45 , 47 , 83 . In addition, depressed patients show impaired inhibition of negative information 7 . As these factors result in a greater share of negative (relative to neutral and positive) information being passed forward for further processing, they likely contribute to additional biases documented at later, more ‘elaborative’ stages of cognition, including preferential recall of negative over positive information 84 , 85 , and biases towards negative interpretations of ambiguous words and word fragments, images (e.g., facial expressions), and scenarios 86 – 88 . Finally, depression has been associated with stronger implicit associations between the mental concept of oneself and negative characteristics, e.g., lower implicit self-esteem, as measured by performance-based indices 89 – 91 . Stronger implicit associations between self and suicide-related constructs (e.g., death) 92 , as well as stronger attentional bias towards suicide-related words 93 , have further been linked to prospective risk of suicide attempts, suggesting a link between affective processing biases and the most severe consequences of depression.
Within the positive valence system, depression involves prominent alterations in the processing of reward cues, such as decreased hedonic pleasure or ‘liking’ of positive stimuli, decreased reward anticipation, and altered reinforcement learning, which are believed to culminate in decreased motivation to act 94 . Recently, computational approaches have been applied in an effort to further dissect these biases and unveil their component neurocomputational processes. Findings from this emerging literature suggest several impairments in reinforcement learning, or the process of maximizing reward and minimizing loss by modifying behavior in response to experience, which plays a central role in decision-making 95 . For instance, depressed patients exhibit both hyposensitivity to rewards and oversensitivity to punishments 96 , 97 , attributed to alterations in mesolimbic prediction error signaling 97 – 99 , and may exhibit a constrained option space (“tunnel vision”) during decision-making 100 . Broadly, positive valence system deficits are linked primarily to altered dopaminergic and/or opioid signaling within midbrain striatal circuits 94 , 101 , but may intersect with plasticity impairments and dysconnectivity of excitatory glutamatergic synapses in an overlapping prefrontal-mesolimbic circuit 5 to produce the full spectrum of affective biases seen in depression.
Though the etiology of these affective information processing biases is not fully understood, the neuroplasticity model of depression suggests a two-fold process: 1) chronic stress, adversity, and negative life events (e.g., parental and/or social interactions) promote learning of negative associations and expectations via normative, experience-dependent plasticity mechanisms, which include evolutionarily preserved mechanisms that prioritize the learning and retention of negative and emotionally salient information 102 , 103 ; 2) such learning is further entrenched through simultaneous, stress-induced decreases in overall plasticity within the prefrontal-limbic circuit, resulting in inflexible, intractable biases that are resistant to subsequent environmental inputs (e.g., positive/disconfirming information). Consistent with our integrative framework ( Table 2 ), the neural substrates of affective biases have been posited to involve inadequate PFC regulation of stimulus-driven limbic responses 8 , 47 and generalized deficiencies of cognitive inhibition 7 . Negative biases in attention 42 , 43 , 104 , 105 and self-representations 44 have been directly linked to reduced functional connectivity in prefrontal-limbic circuitry, suggesting these neural and behavioral features of depression could reflect a unitary plasticity impairment, expressed across levels of analysis. Biased patterns of information processing might then constitute a final pathway to negative affect and self-reported symptomatology (discussed further below), via their chronic and cumulative influence on the individual’s day-to-day perceptions and experiences 82 , 106 – 109 . Further integrative research is needed to evaluate this multi-domain hypothesis.
Summary of key findings supporting integrative neuroplasticity model
Plasticity impairments in self-reported psychological symptoms
Though depression is a highly heterogeneous and complex syndrome, it is notable that the clinical phenomenology of depression includes multiple prominent markers of inflexible thought and behavior, which are broadly consistent with a decreased capacity for the brain to flexibly adjust and reorganize itself in response to a changing environment 6 . The two hallmark mood symptoms of depression—at least one of which is required to diagnose a major depressive episode 110 —are persistent dysphoric mood and persistent anhedonia (the inability to experience pleasure), mirroring the two major forms of information processing bias (positive and negative valence systems) discussed above. Rumination, a form of perseverative negative thought pattern, is a prominent risk factor for depression 111 . Similar perseverative thought patterns, such as worry, ruminative post-event processing, and obsessional thinking, are transdiagnostic features of other (often comorbid) negative affective conditions, leading to the hypothesis that repetitive negative thinking is a core, cross-cutting feature of disorders of negative affect 112 . Depressive “schemas,” the principle treatment target in gold-standard cognitive-behavioral interventions 113 , are likewise characterized by rigid, over-generalized negativity with regard to perceptions of self, future, and the world 9 . Efficacious cognitive-behavioral treatments therefore focus on the goal of expanding plasticity within the perceptions and conscious representations of the patient’s internal and external worlds, through repeated, deliberate practice in recognizing and correcting maladaptive, excessively negative thought patterns 114 . Mindfulness-based cognitive therapy, which can effectively forestall the return of depression among individuals with a depression history 115 , 116 , has similarly been characterized as an effort to increase mental flexibility in response to the environment, specifically through the practice of “de-centering,” or learning to perceive one’s thoughts, feelings and reactions from an objective, non-judgmental, and accepting stance 117 .
The behavioral repertoire reported by depressed patients likewise lacks flexibility and diversity, characterized by symptoms of social withdrawal, behavioral deactivation, lassitude, ‘vegetative symptoms’ (e.g., loss of appetite and libido), and amotivation 110 , 118 , 119 . Depressed patients report that a constrained set of possible actions appear viable, paralyzing action and decision-making 120 . Behavioral activation, a psychotherapy that aims to directly increase activity, can effectively treat depression through a concerted effort to expand on points of contact between the patient and their environment, thereby stimulating natural opportunities for positive reinforcement to be received 119 , 121 , 122 . Thus, plasticity and diversification within the realm of volitional actions may likewise be antithetical to the state of depression.
Testing predictions of the integrative model: Intravenous ketamine as a test case
Our integrative model predicts the falsifiable hypothesis that an agent that reverses molecular neuroplasticity deficits in animal models should exhibit not only clinical effects on depression, but also correlated, simultaneous reversals of impairments at each level of analysis ( Table 1 ). While some of these markers may be quantifiable in animal models, allowing for greater experimental controls (e.g., neural networks; cognition), others may be unique to humans (e.g., information processing; self-report). The use of a rapid-acting agent may be particularly beneficial in human studies, because the influence of many environmental and situational confounds inherent to human research are temporally contained due to the rapidity of ketamine’s effects. However, ketamine research, like most pharmacological research, has focused largely on (a) molecular effects in animal models or (b) symptom-level effects in human patients. While molecular neuroplasticity effects are well-described in rodent models, attempts to extend molecular findings to humans (e.g., BDNF and synapse levels) have yielded inconsistent results 3 , in no small part because of the difficulty of assessing these endpoints in the living brain, the tissue of interest. The cognitive, information processing, and neural network domains offer relatively untapped opportunities to integratively understand how enhanced neuroplasticity might ultimately translate to a profound shift in human experience, i.e. rapid depression relief.
Preliminary findings on ketamine’s effects on neurocognition are consistent with the proposed model of neuroplasticity, suggesting clues as to how ketamine’s rapid clinical and neuronal/molecular effects might manifest in other domains.
Neural networks:
fMRI investigations in depressed patients have linked ketamine’s antidepressant effects to increased connectivity between mPFC and striatum 123 , between lateral PFC and subgenual ACC 124 , and to increased global connectivity between the PFC and the rest of the brain 55 . Convergent data show increased PFC glucose metabolism 24-hours post-ketamine 125 , 126 , increased fMRI PFC activations acutely (during infusion itself) 127 , and decreased limbic responses to angry (relative to happy) faces among ketamine responders 128 . Increased connectivity between the default mode network (DMN), which encompasses the medial PFC, and the insula have also been reported in depressed patients 2 days following ketamine, suggesting sustained normalization of the DMN’s relationship to other networks 129 .
Cognitive function:
Two studies in rats 130 , 131 extend molecular findings in animal models to behavioral indices with possible relevance to cognitive flexibility in human patients. Following stress, rats randomized to ketamine (compared to saline) exhibited enhanced cognitive flexibility, as indexed by improved set-shifting task performance. In small, uncontrolled samples, convergent findings in depressed patients further suggest cognitive flexibility on objective cognitive tests improves acutely following ketamine 132 , 133 .
Affective information processing:
Ketamine rapidly induces flexibility in implicit representations of self on the Implicit Association Test 134 , 135 , and these shifts correlated with self-reported symptom improvements 110 , suggesting that ketamine can immediately impact the structure of implicit mental representations relevant to one’s concept of self, a core form of cognitive bias in depression. Although in a separate study, ketamine failed to illustrate a similar impact on attentional bias towards negative cues 128 , the use of a reaction time measure (the dot-probe task) with notoriously poor psychometric properties 136 – 138 may have impeded the ability to sensitively detect changes over time.
Further integration across multiple markers of plasticity is needed to build support for an integrative model, as highlighted by the small number of direct “integration” links in Table 2 . Of note, to accurately test for relationships in individual differences expressed across patients with correlational and mediational investigations, larger sample sizes are likely required relative to those necessary simply to establish the antidepressant efficacy of ketamine. Ongoing work in depression (e.g., R01MH113857;) aims to comprehensively characterize and link ketamine’s depression-relevant effects across molecular, neural network, cognitive, implicit processing, and symptomatic levels of analysis in human patients, in the hopes of establishing a multi-domain neuroplasticity signature.
Testing predictions of the integrative model: Experimental manipulation at each level
In addition to manipulating molecular neuroplasticity targets (e.g., pharmacologically), a complimentary approach is to evaluate the causal influence of each posited form of plasticity by manipulating it directly (e.g., through mechanistic intervention; see Table 2 :’Causality’ for specific examples and evidence base) and observing its influence on clinical depression and all other levels of analysis. Leveraging RCT designs and explicit tests of ‘target engagement’ (to validate that the targeted neural, cognitive, or information processing manipulation was successful), this approach has the potential to distinguish causal influences on depression from depression correlates and to build support for a causal chain of neuroplasticity decrements leading to depression symptoms.
Generalizability and Transdiagnostic Relevance
Though the current review focuses on depression, it is notable that chronic stress is a risk factor for virtually all psychiatric conditions 139 . Likewise, the neural, cognitive, information processing, and psychological patterns discussed above have broad transdiagnostic relevance, particularly with respect to other disorders of negative affect (e.g., anxiety, trauma-related conditions) 140 —that likewise respond to pharmacologic interventions with plasticity-enhancing properties 141 , 142 . Equally noteworthy, however, is the marked heterogeneity of depressed patients, which is evident at the neural network 143 , 144 , cognitive 145 , information processing 145 , 146 , and clinical 147 levels, and may help to explain the fact that no known intervention strategy is effective for all depressed patients. Rather than assuming a unitary etiology, and a correspondingly uniform pathway to recovery from depression, a focus on establishing correlations across levels of analysis—both before and after administering plasticity-enhancing interventions—will help to clarify the degree to which an integrative neuroplasticity model may be relevant to some individual forms of depression and negative affect, but not others 140 .
Neuroplasticity, or the brain’s capacity to flexibly adjust and reorganize itself in response to a changing environment, is a fundamental contributor to adaptive functioning. Impairments of neuroplasticity often characterize disorders of negative affect including depression 3 , 4 and multiple efficacious therapies often reverse such deficits 10 , 148 – 151 . Our review highlights conceptual convergence of findings across relatively disparate literatures in basic neuroscience, cognitive science, and clinical psychology. Direct empirical links remain quite preliminary ( Table 2 ). We hope to stimulate future studies with a broader integrative focus to empirically elucidate the intermediary mechanisms that allow neuronal and molecular changes at the cellular level to propagate up through a complex, circuit-based neurocognitive system. Ideally this work will suggest new treatment avenues to provide relief from pervasive, chronic, inflexible, and debilitating patterns of mood and behavior. Such novel treatments might aim, for example, to synergistically target more than one form of plasticity deficit simultaneously through theory-driven, somatic-behavioral treatment pairings 152 .
Acknowledgements:
This project was supported in part by National Institute of Mental Health grant number R01MH113857 (RBP).
Conflict of Interest: Dr. Price and Dr. Duman report no conflicts of interest or competing financial interests.
Thank you for visiting nature.com. You are using a browser version with limited support for CSS. To obtain the best experience, we recommend you use a more up to date browser (or turn off compatibility mode in Internet Explorer). In the meantime, to ensure continued support, we are displaying the site without styles and JavaScript.
- View all journals
- My Account Login
- Explore content
- About the journal
- Publish with us
- Sign up for alerts
- Open access
- Published: 04 April 2024
Patterns of stressful life events and polygenic scores for five mental disorders and neuroticism among adults with depression
- Jacob J. Crouse ORCID: orcid.org/0000-0002-3805-2936 1 ,
- Shin Ho Park 1 ,
- Enda M. Byrne ORCID: orcid.org/0000-0002-9491-7797 2 , 3 ,
- Brittany L. Mitchell ORCID: orcid.org/0000-0002-9050-1516 4 ,
- Jan Scott ORCID: orcid.org/0000-0002-7203-8601 1 , 5 , 6 , 7 ,
- Sarah E. Medland ORCID: orcid.org/0000-0003-1382-380X 4 ,
- Tian Lin 2 ,
- Naomi R. Wray ORCID: orcid.org/0000-0001-7421-3357 2 , 8 , 9 , 10 ,
- Nicholas G. Martin ORCID: orcid.org/0000-0003-4069-8020 4 &
- Ian B. Hickie ORCID: orcid.org/0000-0001-8832-9895 1
Molecular Psychiatry ( 2024 ) Cite this article
Metrics details
The dominant (‘general’) version of the diathesis-stress theory of depression views stressors and genetic vulnerability as independent risks. In the Australian Genetics of Depression Study ( N = 14,146; 75% female), we tested whether polygenic scores (PGS) for major depression, bipolar disorder, schizophrenia, anxiety, ADHD, and neuroticism were associated with reported exposure to 32 childhood, past-year, lifetime, and accumulated stressful life events (SLEs). In false discovery rate-corrected models, the clearest PGS-SLE relationships were for the ADHD- and depression-PGSs, and to a lesser extent, the anxiety- and schizophrenia-PGSs. We describe the associations for childhood and accumulated SLEs, and the 2–3 strongest past-year/lifetime SLE associations. Higher ADHD-PGS was associated with all childhood SLEs (emotional abuse, emotional neglect, physical neglect; ORs = 1.09–1.14; p’s < 1.3 × 10 − 5 ), more accumulated SLEs, and reported exposure to sudden violent death (OR = 1.23; p = 3.6 × 10 − 5 ), legal troubles (OR = 1.15; p = 0.003), and sudden accidental death (OR = 1.14; p = 0.006). Higher depression-PGS was associated with all childhood SLEs (ORs = 1.07–1.12; p’s < 0.013), more accumulated SLEs, and severe human suffering (OR = 1.17; p = 0.003), assault with a weapon (OR = 1.12; p = 0.003), and living in unpleasant surroundings (OR = 1.11; p = 0.001). Higher anxiety-PGS was associated with childhood emotional abuse (OR = 1.08; p = 1.6 × 10 − 4 ), more accumulated SLEs, and serious accident (OR = 1.23; p = 0.004), physical assault (OR = 1.08; p = 2.2 × 10 − 4 ), and transportation accident (OR = 1.07; p = 0.001). Higher schizophrenia-PGS was associated with all childhood SLEs (ORs = 1.12–1.19; p’s < 9.3 − 8 ), more accumulated SLEs, and severe human suffering (OR = 1.16; p = 0.003). Higher neuroticism-PGS was associated with living in unpleasant surroundings (OR = 1.09; p = 0.007) and major financial troubles (OR = 1.06; p = 0.014). A reversed pattern was seen for the bipolar-PGS, with lower odds of reported physical assault (OR = 0.95; p = 0.014), major financial troubles (OR = 0.93; p = 0.004), and living in unpleasant surroundings (OR = 0.92; p = 0.007). Genetic risk for several mental disorders influences reported exposure to SLEs among adults with moderately severe, recurrent depression. Our findings emphasise that stressors and diatheses are inter-dependent and challenge diagnosis and subtyping (e.g., reactive/endogenous) based on life events.
Introduction
The diathesis-stress model is the dominant aetiological theory of depressive disorders [ 1 ]. Multiple versions of this model have been proposed, which each describe different forms of relationship between stressors and diatheses and have distinct implications for understanding, preventing, and treating depression [ 2 ]. While several studies support a complex, inter-dependent relationship between stressors and diatheses [ 3 , 4 ], the ‘general’ version of the model (which predicts that people with a higher genetic vulnerability for depression should have a lower load of stressors compared to people with lower genetic vulnerability) remains the most popular theory of the aetiology of depression [ 5 ]. Specific typologies of depression have been proposed based on this idea [ 6 ]. For example, people with so-called ‘endogenous’ depression have been theorised to have high genetic or biologic load but low environmental load, while cases with ‘reactive’ depression are theorised to have low genetic or biologic load but high environmental load.
The general version of the diathesis-stress model of depression and the proposed distinction between ‘endogenous’ and ‘reactive‘ subtypes fail to adequately consider that stressors and genetic diatheses are often inter-related. Indeed, there is some evidence that exposure to SLEs may be partly heritable [ 7 ], with twin studies showing that a class of SLEs for which individuals might play a contributory role seem to be influenced by genetic factors, albeit with modest heritability estimates [ 8 , 9 , 10 , 11 ]. This class of SLEs are referred to as ‘dependent’ or ‘personal’ SLEs (e.g., being laid off from one’s job), while SLEs that are more likely to be a function of random chance (e.g., exposure to a natural disaster) are referred to as ‘independent’ or ‘nonpersonal’ SLEs. Three models are commonly used to explain such ‘gene-environment correlations’. The ‘active’ model views people with genetic vulnerability to depression as being more likely to generate stress by selecting themselves into environments that have higher risk of exposure to stressors, such as a dysfunctional intimate relationship [ 12 , 13 , 14 , 15 ]. By contrast, the ‘passive’ model suggests that since mental disorders are heritable, genetic vulnerability may be a (noncausal) marker of a parent’s psychopathology, and a parent’s mental health problems may be the proximal cause of increased risk of exposure to SLEs in offspring. Third, the ‘evocative’ model proposes that genetically-influenced traits (e.g., stubbornness) evoke predictable patterns of response from the social environment (e.g., berated by a family member). Given these theoretical distinctions and the plausible contribution of individual factors (e.g., genes) to reported exposure to SLEs, a coherent aetiologic theory of depression should consider the influence genes have on exposure (or reporting of exposure) to SLEs [ 16 ].
Recent genetic studies have tested whether people with a higher polygenic score (PGS) for depression are more likely to develop depression after exposure to SLEs, with conflicting findings [ 3 , 4 , 17 , 18 , 19 ]. Very few studies have examined whether PGSs for depression or other mental disorders (and correlated traits) are associated with reported exposure to SLEs. A study of cases with depression in iPSYCH2012 reported a small relationship between higher depression-PGS and increased risk of exposure to at least one SLE after age 10 18 . A case-control study reported a small association between higher depression-PGS and the number of SLEs individuals were exposed to, but only in cases with depression [ 20 ]. Population-based studies have also investigated this question. A study from Generation Scotland reported a small association between a higher depression-PGS and the number of reported SLEs [ 21 ]. By contrast, a study of older adults in the Health and Retirement Study reported that the depression-PGS was unrelated to exposure to SLEs [ 22 ]. A handful of studies have examined whether multiple PGSs for mental disorders are mutually associated with reporting of SLEs. A study of a population-based cohort of women found that higher PGSs for major depression and ADHD were associated with higher likelihood of reporting exposure to childhood abuse, while PGSs for neuroticism, schizophrenia, bipolar disorder, and autism spectrum disorder (ASD) were associated with physical and emotional (but not sexual) abuse [ 23 ]. A study of the Twins Early Development Study reported a higher likelihood of retrospective childhood trauma in those with higher PGSs for ASD and PTSD, but not major depression, ADHD, bipolar disorder, schizophrenia, neuroticism, or anxiety [ 24 ].
No study of people with depression has investigated the mutual impact of PGSs for depression and other mental disorders on reported exposure to SLEs. Accordingly, we use a cohort study of adults with depression to examine associations between reported exposure to SLEs and PGSs for five major mental disorders (depression, bipolar disorder, schizophrenia, anxiety disorder, ADHD) and a related trait (neuroticism). We focus on these traits for four reasons. First, they are relevant to diathesis-stress models of depression and have plausible links to the experience, reporting, and/or exposure to SLEs (particularly PGS for neuroticism, anxiety, and depression). Second, there is face validity in the idea that behavioural manifestations of genetic liability to these six traits may increase the likelihood of being exposed to SLEs (particularly PGS for schizophrenia and ADHD). Third, these PGS are relatively well-powered, with SNPs identified by suitably-powered GWAS. Fourth these six traits are central to our ‘tripartite’ model about differential pathways to depressive disorders [ 25 , 26 ]. Building on the literature, we hypothesise that higher depression-PGS will be associated with higher likelihood of reported exposure to SLEs, while investigation of the other PGS-SLE associations are exploratory.
Materials and methods
Participants and study design.
The Australian Genetics of Depression Study (AGDS) is a volunteer cohort study of adults with depression. Recruitment procedures and cohort characteristics have been detailed elsewhere [ 27 ]. Participants joined AGDS after replying to a media campaign or a letter from the Australian Government’s Department of Human Services, sent to Australian residents who had received ≥ 4 prescriptions of any of the 10 commonest antidepressant medications in Australia in the past 4.5 years. 100,000 letters were sent across two waves (2016, 2017) and more participants were recruited via media appeal (85.7%) than prescription history invitation (14.3%). Participants completed an online survey about mental/physical health, treatment, and social, behavioural, and environmental factors [ 27 ]. The sample are highly educated (32% with a degree, 24% with a postgraduate degree) and > 75% have contributed a saliva sample using a mail-out ‘spit-kit’ from which DNA was extracted and processed.
The study was approved by the QIMR Berghofer Medical Research Institute Human Research Ethics Committee in Brisbane, Australia. Written informed consent was obtained from all participants. This report followed the Strengthening the Reporting of Observational Studies in Epidemiology (STROBE) guidelines [ 28 ].
Stressful life events (SLEs)
Childhood sles.
Participants indicated whether they experienced any of three types of SLEs in childhood: (1) emotional abuse (e.g., often being told you were no good; yelled at in a scary way; threatened, ignored, or stopped from making friends); (2) emotional neglect (e.g., often not being shown affection; not being given encouragement or support); or (3) physical neglect (e.g., often not being given enough to eat or drink, appropriate clothing, shelter, medical care, education, supervision, or a safe home environment). Three possible responses (no, yes, unsure) were recoded (1 = yes; 0 = no/unsure).
Lifetime SLEs
Estimated using the Life Events Checklist for DSM-5 [ 29 ]. Participants indicated whether they had experienced any of the following SLEs: (1) natural disaster (e.g., flood, cyclone, tornado, earthquake); (2) fire or explosion; (3) transportation accident (e.g., car accident, boat accident, train wreck, plane crash); (4) serious accident at work, home, or during recreational activity; (5) exposure to toxic substances (e.g., dangerous chemicals, radiation); (6) physical assault (e.g., being attacked, hit, slapped, kicked, beaten up); (7) assault with a weapon (e.g., being shot, stabbed, threatened with a knife, gun, bomb); (8) sexual assault (e.g., rape, attempted rape, made to perform any type of sexual act through force or threat of harm); (9) other unwanted/uncomfortable sexual experience; (10) combat or exposure to a war-zone (in the military or as a civilian); (11) captivity (e.g., being kidnapped, abducted, held hostage, prisoner of war); (12) life-threatening illness or injury; (13) severe human suffering; (14) sudden violent death (e.g., homicide, suicide); (15) sudden accidental death; (16) serious injury, harm or death you caused to someone else; or (17) any other very stressful event or experience. Six possible responses (happened to me, witnessed it, learned about it, part of my job, not sure, doesn’t apply) were recoded (1 = happened to me; 0 = all other responses).
Past-year SLEs
Estimated using 12 items adapted from the List of Threatening Experiences [ 30 ]. Participants indicated whether they experienced any of the following in the past 12 months: (1) divorce; (2) marital separation; (3) broken engagement/steady relationship; (4) separation from other loved one or close friend; (5) serious illness or injury; (6) serious accident (not involving personal injury); (7) burgled or robbed; (8) laid off or sacked from job; (9) other serious difficulties at work; (10) major financial problems; (11) legal troubles or involvement with police; or (12) living in unpleasant surroundings. Two responses were possible (0 = no; 1 = yes).
Cumulative SLEs
We summed the number of SLEs across four categories: childhood (range = 0–3); lifetime (0–17); past-year (0–12); and overall (i.e., summing across the other three categories; 0–32). Four SLEs had conceptual overlap across the checklists: ‘serious accident’ (lifetime) with ‘serious accident’ (past-year) and ‘life-threatening illness or injury’ (lifetime) with ‘serious illness or injury’ (past-year). To avoid double-counting, we discarded the past-year version when creating the cumulative SLE variable.
Polygenic scores (PGS)
Genotyping was done using the Illumina Global Screening Array V2. Samples were merged with the 1000 Genomes project samples [ 31 ] and genetic principal components (PCs) were calculated using a set of single-nucleotide polymorphisms (SNPs) not in linkage disequilibrium. Pre-imputation quality control was done using PLINK 1.9 [ 32 , 33 ], including removing SNPs with a minor allele frequency < 0.005, SNP call rate < 97.5%, and identification of participants with genetic similarity [ 34 ] to a European reference group (> 4 SD from Ancestry Principal Components [PCs] PC1/PC2 centroid) and Hardy-Weinberg equilibrium ( p < 1 × 10 − 6 ), before imputation using the Haplotype Reference Consortium 1.1 reference panel [ 35 ]. Over 95% of AGDS is of European ancestry and PGS were created for those with European ancestry only. PCA plots of ancestry principal components are shown in Supplementary Fig. 1 . Other than requiring data for PGS and SLEs, no inclusion/exclusion criteria was applied for this study.
Summary statistics from recent genome-wide association studies (GWAS) were used to identify SNPs associated with major depression [ 36 ], bipolar disorder [ 37 ], schizophrenia [ 38 ], anxiety [ 39 ], ADHD [ 40 ], and neuroticism [ 41 ]. SBayesR [ 42 ], a Bayesian PGS method, was used to generate allele weights for each PGS. The posterior SNP effects for each disorder/trait were used to generate PGS for each participant using the PLINK score function [ 32 ]. As SNPs from AGDS participants have been used in depression GWAS, our depression-PGS was calculated with AGDS participants excluded. Each PGS was standardised across the entire QIMR genetic datasets ( N > 60,000); the mean and standard deviations (SD) of each PGS are: major depression ( M = 0.29; SD = 0.98); bipolar disorder ( M = 0.11; SD = 1.00); schizophrenia ( M = 0.07; SD = 1.00); anxiety disorder ( M = 0.12; SD = 0.99); ADHD ( M = 0.07; SD = 1.00); and neuroticism ( M = 0.10; SD = 0.96). PGS were standardised for analysis. Effect sizes are interpretable as reflecting a one SD increase/decrease.
Statistical analysis
Analyses were conducted using R (version 4.2.2) [ 43 ]. Pearson’s product moment correlation was used to examine univariate relationships between each PGS (continuous) and the 32 SLEs (dichotomous). Logistic regression was used to model associations between SLEs and PGSs, while linear regression was used to model associations between the number of SLEs in each epoch (childhood, lifetime, past-year) and accumulated (range 0–32) and the PGS; models were adjusted for age and sex. The data were inspected before running analyses to confirm they met the assumptions of the statistical tests. The sample size was not pre-determined for this specific study but reflects participants with data on PGS and SLEs. To control for multiple comparisons in the multivariate analyses, we report uncorrected p -values and false discovery rate (FDR) significant p -values, calculated using the Benjamini-Hochberg (BH) procedure [ 44 ]: (1) sort p -values from multivariable models in ascending order; (2) assign a rank to each p -value; (3) calculate each p -value’s BH critical value using the formula (i/m)Q, where: i = rank of p -value, m = number of tests, and Q = false discovery rate (5%); and (4) find largest p -value that is less than the critical value and designate smaller p -values as “significant”. Model outputs are reported in Supplementary Tables 5 – 40 .
Demographic and clinical data
From an available cohort of 20,680 individuals (75% female; mean [SD] age 42.8 years [15.3 years]), data passing quality control were available for 14,146 participants and included as our analytic sample. 75% were female and the mean [SD] age was 44.0 years [15.3] (range 18–90). Using DSM-5 criteria, 88% met criteria for a lifetime major depressive episode. Descriptive information about the sample is provided in Table 1 .
Reported exposure to SLEs
Numbers of participants reporting exposure to each of the 32 SLEs are presented in Supplementary Table 1 . The three commonest were emotional abuse (53%) and emotional neglect (46%) in childhood and lifetime other unwanted or uncomfortable sexual experience (34%), while the least common was combat or exposure to a warzone (< 1%).
Childhood SLEs and PGSs
As shown in Fig. 1 , higher PGSs for ADHD, schizophrenia, and depression were associated with a higher likelihood of reported exposure to all childhood SLEs (FDR-corrected p’s < 0.05). Higher anxiety-PGS was associated with higher odds of reported exposure to emotional abuse in childhood (FDR-corrected p < 0.05). The bipolar- and neuroticism-PGS were not associated with reported exposure to any childhood-SLE.
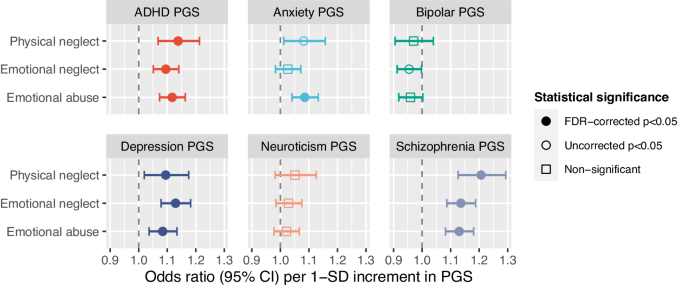
Coefficients are presented grouped by PGS (not SLE). The y -axis variables are the outcome (y variables) for three regression models in which the six PGS were fitted alongside age and sex (x variables).
Lifetime SLEs and PGSs
As shown in Fig. 2 , under FDR-correction there were no significant relationships between any lifetime-SLE and the neuroticism-PGS. The bipolar-PGS was associated a lower likelihood of reported exposure to physical assault (OR = 0.95 [95% CI,0.91–0.99]; p = 0.014; FDR-corrected p < 0.05). Higher schizophrenia-PGS was related to a higher odds of severe human suffering (OR = 1.16 [1.05–1.29]; p = 0.003; FDR-corrected p < 0.05). Higher anxiety-PGS was associated with higher likelihood of physical assault (OR = 1.08 [1.04–1.13]; p < 0.001; FDR-corrected p < 0.05) and transportation accident (OR = 1.07 [1.03–1.11]; p = 0.001; FDR-corrected p < 0.05). Higher depression-PGS was associated with higher odds of physical assault (OR = 1.06 [1.02–1.11]; p = 0.006; FDR-corrected p < 0.05), unwanted/uncomfortable sexual experience (OR = 1.09 [1.04–1.13]; p < 0.001; FDR-corrected p < 0.05), sexual assault (OR = 1.10 [1.05–1.16]; p < 0.001; FDR-corrected p < 0.05), severe human suffering (OR = 1.17 [1.05–1.30]; p = 0.003; FDR-corrected p < 0.05), life-threatening illness or injury (OR = 1.09 [1.03–1.15]; p = 0.003; FDR-corrected p < 0.05), and assault with a weapon (OR = 1.12 [1.04–1.21]; p = 0.003; FDR-corrected p < 0.05). Finally, higher ADHD-PGS was associated with higher likelihood of sudden violent death (OR = 1.23 [1.12–1.36]; p < 0.001; FDR-corrected p < 0.05), sudden accidental death (OR = 1.14 [1.04–1.25]; p = 0.006; FDR-corrected p < 0.05), sexual assault (OR = 1.11 [1.07–1.16]; p < 0.001; FDR-corrected p < 0.05), serious accident (OR = 1.08 [1.03–1.14]; p = 0.004; FDR-corrected p < 0.05), physical assault (OR = 1.09 [1.05–1.14]; p < 0.001; FDR-corrected p < 0.05), fire or explosion (OR = 1.11 [1.04–1.19]; p = 0.001; FDR-corrected p < 0.05), assault with a weapon (OR = 1.11 [1.04–1.19]; p = 0.001; FDR-corrected p < 0.05), and transportation accident (OR = 1.05 [1.01–1.09]; p = 0.018; FDR-corrected p < 0.05).
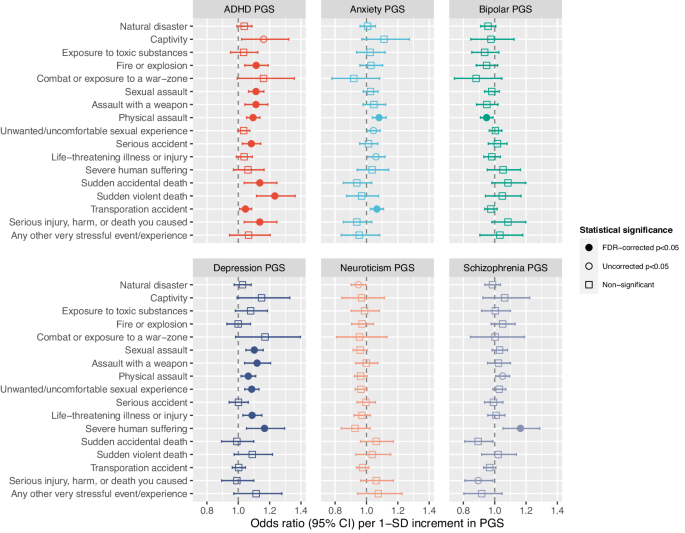
Coefficients are presented grouped by PGS (not SLE). The y -axis variables are the outcome ( y variables) for 17 regression models in which the six PGS were fitted alongside age and sex (x variables).
Past-year SLEs and PGSs
As presented in Fig. 3 , under FDR-correction the schizophrenia-PGS was not significantly associated with any past-year SLE. Higher anxiety-PGS was associated with a higher likelihood of serious accident (OR = 1.23 [95% CI,1.07–1.42]; p = 0.004; FDR-corrected p < 0.05). Higher neuroticism-PGS was associated with higher likelihood of living in unpleasant surroundings (OR = 1.09 [1.02–1.15]; p = 0.007; FDR-corrected p < 0.05) and major financial troubles (OR = 1.06 [1.01–1.12]; p = 0.014; FDR-corrected p < 0.05). Higher depression-PGS was associated with higher odds of serious illness or injury (OR = 1.09 [1.04–1.15]; p < 0.001; FDR-corrected p < 0.05), major financial troubles (OR = 1.08 [1.03–1.14]; p = 0.002; FDR-corrected p < 0.05), and living in unpleasant surroundings (OR = 1.11 [1.04–1.18]; p = 0.001; FDR-corrected p < 0.05). By contrast, higher bipolar-PGS was associated lower likelihood of major financial troubles (OR = 0.93 [0.88–0.98]; p = 0.004; FDR-corrected p < 0.05) and living in unpleasant surroundings (OR = 0.92 [0.87–0.98]; p = 0.008; FDR-corrected p < 0.05). Finally, higher ADHD-PGS was associated with higher odds of serious illness or injury (OR = 1.10 [1.05–1.16]; p < 0.001; FDR-corrected p < 0.05), separation from loved one (OR = 1.08 [1.03–1.14]; p = 0.002; FDR-corrected p < 0.05), major financial troubles (OR = 1.11 [1.06–1.17]; p < 0.001; FDR-corrected p < 0.05), and legal troubles (OR = 1.15 [1.05–1.25]; p = 0.003; FDR-corrected p < 0.05).
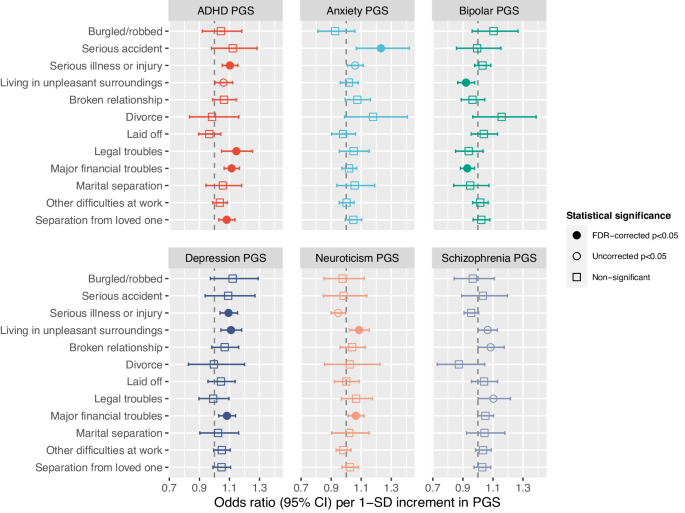
Coefficients are presented grouped by PGS (not SLE). The y -axis variables are the outcome ( y variables) for 12 regression models in which the six PGS were fitted alongside age and sex ( x variables).
Cumulative SLEs and PGSs
As shown in Fig. 4 , the neuroticism-PGS was not significantly related to any cumulative SLE variable. The schizophrenia-PGS was related to more SLEs in childhood, the lifetime, and overall (ORs = 1.09–1.20; FDR-corrected p’s < 0.05). Higher ADHD-PGS was related to more childhood, lifetime, past-year, and cumulative SLEs (ORs = 1.07–1.36; FDR-corrected p’s < 0.05), as was the depression-PGS (ORs = 1.05–1.24; FDR-corrected p’s < 0.05) and anxiety-PGS (OR = 1.04–1.13; FDR-corrected p’s < 0.05). By contrast, higher bipolar-PGS was related to fewer reported SLEs in childhood (OR = 0.97 [0.95–0.99]; p = 0.01; FDR-corrected p < 0.05).
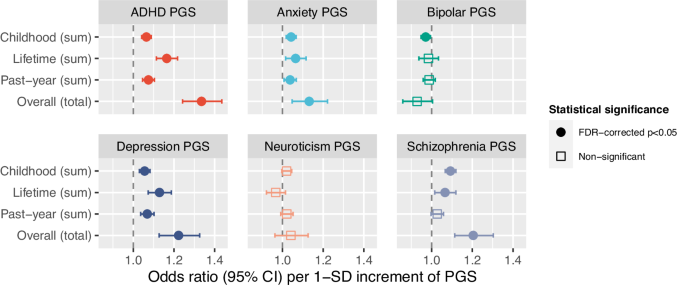
For simplicity, coefficients are presented grouped by PGS (not SLE). The y -axis variables are the outcome ( y variables) for four separate regression models in which the six PGS were fitted alongside age and sex (x variables).
Sensitivity analyses
Depressive episodes.
Higher depression-PGS is associated with more depressive episodes; people experiencing more episodes may be more likely to be subsequently exposed to SLEs; and more episodes may lead to hopelessness that biases reporting. The depression-, schizophrenia-, anxiety-, neuroticism-, and ADHD-PGS were each associated with more depressive episodes and more SLEs, and the number of depressive episodes was associated with all childhood and most past-year and lifetime SLEs (Supplementary Tables 2 – 4 ). When including number of depressive episodes as a covariate, the PGS-SLE associations were slightly attenuated but qualitatively similar (Supplementary Tables 5 – 40 ).
Non-psychiatric PGS
It is possible that the genetic relationships with SLEs are not specific to psychiatric PGS. In a post-hoc analysis, we chose six control PGS, three with minimal genetic correlations (rG = 0.02 0.04) with depression (hip circumference, rheumatoid arthritis, type 2 diabetes [T2D]) and three with stronger genetic correlations (rG = 0.15–0.16) with depression (lung cancer, asthma, coronary artery disease [CAD]). These non-psychiatric PGS were standardised against an independent control sample (ASPREE). We ran the same regression models but with the psychiatric PGS removed and the ‘control’ PGS fitted together (Supplementary Figs. 2 – 5 ).
Starting with the traits more strongly genetically correlated with depression, the lung cancer-PGS was associated with three SLEs: ‘serious illness or injury’ (OR = 1.06; p = 0.012); major financial problems (OR = 1.06; p = 0.008); and sudden violent death (OR = 1.11; p = 0.036). The asthma-PGS was associated with eight SLEs, with the five strongest associations for: count of accumulated SLEs (OR = 1.12; p = 0.002); major financial problems (OR = 1.08; p = 0.011); living in unpleasant surroundings (OR = 1.07; p = 0.016); unwanted sexual experience (OR = 1.06; p = 0.004); and physical assault (OR = 1.06; p = 0.007). The CAD-PGS was associated with six SLEs, with the five strongest associations for: serious injury, harm, or death you caused to someone else (OR = 1.17; p = 0.014); legal troubles or involvement with police (OR = 1.14; p = 0.003); count of accumulated SLEs (OR = 1.09; p = 0.016); count of lifetime SLEs (1.06; p = 0.016); and serious illness or injury (OR = 1.06; p = 0.020).
Regarding the traits minimally correlated with depression, the hip circumference-PGS was associated with three reported SLEs: fire or explosion (OR = 0.93; p = 0.037); unwanted sexual experience (OR = 0.96; p = 0.041); and harm you caused (OR = 1.13; p = 0.046). The rheumatoid arthritis-PGS was associated with two reported SLEs: fire or explosion (OR = 0.90; p = 0.002) and serious injury, harm, or death you caused to someone else (OR = 1.14; p = 0.033). Finally, the T2D-PGS was associated with 18 SLE variables, with the strongest five associations being: marital separation (OR = 1.21; p = 0.001); total accumulated SLEs (OR = 1.18; p < 0.001); harm you caused (OR = 1.18) ( p = 0.007); sexual assault (OR = 1.09; p < 0.001); and major financial problems (OR = 1.09; p = 0.001).
In this cohort study of adults with depression, genetic vulnerability for several mental disorders was associated with an increased likelihood of reported exposure to SLEs. While all PGSs were associated with SLEs to varying degrees, the clearest patterns of increased likelihood were for depression and ADHD, and, to a lesser extent, schizophrenia and anxiety. By contrast, higher genetic risk for bipolar disorder was associated with lower odds of reported exposure to several SLEs, consistent with one study finding a lower bipolar-PGS among cases with more childhood maltreatment [ 45 ] but inconsistent with another reporting the opposite [ 46 ]. While counterintuitive, this association does not mean that genetic vulnerability to BD reduces the risk of genuine exposure to SLEs; rather, genetic vulnerability to BD might influence the way that individuals think about and respond to questions about stressors. These findings underscore the inter-dependence of diatheses and stressors in depression and also challenge the historical discourse about the subtyping of depression on the basis of exposure to SLEs (e.g., melancholic vs non-melancholic; endogenous vs reactive). The findings also bring into question the role of SLEs and other perceived traumatic experiences in diagnostic categories that rely primarily on the reporting of events (e.g., PTSD, prolonged grief disorder). While these experiences may represent a precipitant of abnormal mental states, the simple assignment of aetiologic causality to reported events may downplay the importance of other vulnerability factors that underpin variation in responses to SLEs. We discuss these findings in the context of active, passive, and evocative gene-environment correlations, and gene-environment interaction.
The active gene-environment correlation account views people with genetic vulnerability to depression as being more likely to generate stress by selecting themselves into environments carrying higher risk of stressors [ 12 , 13 , 14 , 15 ]. In support of this, some studies show that genetic risk for depression is more strongly linked to interpersonal than nonpersonal SLEs. Chance is presumed to play a more prominent causal role in nonpersonal SLEs [ 4 , 13 , 20 , 47 ]. Here, most of the positive PGS-SLE associations were for personal SLEs (e.g., legal problems, sexual assault, physical assault). Dovetailing with other studies, the depression-PGS was mostly associated with personal SLEs [ 4 ], and notably, the ADHD-PGS was related to the highest number of personal SLEs. Conversely, the passive account suggests that since mental disorders are heritable, genetic vulnerability may be a (noncausal) marker of a parent’s psychopathology. Parental psychopathology could therefore be a cause of an offspring’s reported exposure to SLEs, for example, via a chaotic home environment [ 48 ] or negative parenting behaviours in childhood [ 49 ]. Third, the evocative account suggests that genetically-influenced traits evoke predictable patterns of response from the environment. Children may express traits (e.g., aggression, headstrong behaviour) that make parents engage in negative behaviours (e.g., emotional abuse). Relevant to our findings, we speculate that behavioural expression of genetic liability to ADHD (e.g., impulsivity) [ 50 ], depression (e.g., moodiness), or schizophrenia (e.g., behavioural disturbance) [ 51 ] could elicit negative responses from the environment, and these responses could be experienced or interpreted as SLEs.
A final interpretation is gene-environment interaction. As has been suggested for genetic risk for depression [ 13 ], genetic risk for other mental disorders (e.g., anxiety) may alter an individual’s sensitivity to the environment. For example, an individual with genetic vulnerability to anxiety may experience an event as stressful or attribute the intention of another person as threatening. Such genetic vulnerability could influence a shift from a neutral event—e.g., being touched on the arm as a greeting—to a stressful event—e.g., feeling physically assaulted. Relatedly, we have proposed a developmental pathway model that hypothesises three pathophysiological mechanisms underpinning trajectories to depression: a neurodevelopmental pathway leading to depression with cognitive impairment or psychotic features; a circadian pathway leading to atypical depression or bipolar-like presentations; and a hyperarousal pathway leading to the common forms of anxious-depression [ 25 , 26 , 52 ]. Speculating about our findings under this model, it is possible that for one person with high genetic risk for depression or anxiety, a neutral event may be experienced with hyperarousal and be recalled as a stressor, while for another person with lower genetic risk for depression or anxiety, the same event might be experienced with lower arousal and not be recalled as a stressor. Age-dependent expression of genetic risk may also be relevant. For example, a study of the Avon Longitudinal Study of Parents and Children reported that higher genetic risk for schizophrenia manifests in adolescence as anxiety [ 53 ]; people with genetic liability to schizophrenia might therefore experience life events as more stressful at certain developmental stages.
Our study has several limitations. First, we relied on retrospective reports of SLEs, which might be influenced by reporting/recall biases. Some SLE items were broad, subject to interpretation, and may reflect non-abusive behaviours; for instance, the childhood ‘emotional abuse’ item includes example prompts such as “yelled at in a scary way” and “not being given encouragement”, which may be particularly at risk of recall biases. Second, it is possible that participants may have conflated their experience of depression with items on the SLE checklist. Third, most of AGDS reports a severe, recurrent course of depression (only 4% reporting a single episode [ 27 ]). While our models adjusted for the number of depressive episodes, our findings might nonetheless be less relevant to individuals with less severe or recurrent depressive disorders. Fourth, we used the full distribution of each PGS; however, given that differences in SLEs could plausibly be quite different at the extremes of the PGS, in an exploratory analysis we compared the ORs for each SLE between the top and bottom 5% of each PGS. Across the six psychiatric PGS, there was a difference of less than 10% in the ORs of most SLEs, which suggests that if there are nonlinear PGS-SLE associations, they are minor. Fifth, we cannot know whether the PGS-SLE associations reflect an increase in the probability of genuine exposure to SLEs versus an increase in the attribution of events as stressors. Sixth, differences in the patterns of association between the PGS may be related to differences in the relative power of each PGS (which are influenced by GWAS sample sizes and the genetic architecture of the phenotypes). Seventh, most of AGDS is of European ancestry (> 95%) and our PGS analyses were on participants with genetic similarity to a European reference group; our adjustment for genetic PCs accounts for differences within the European group and cryptic relatedness between samples (which in one sense is a strength). However, our findings may not be generalisable to non-European groups, as reporting of SLEs (and associations with PGS) might differ across ancestry/ethnic groups. Eighth, the higher proportion of participants recruited to AGDS via public media appeal (86%) compared to prescription history invitation (14%), and the high educational attainment, may indicate a self-selection bias that restricts the generalisability of our findings. Ninth, a person’s current relationship with family could bias their reporting of childhood SLEs. The survey did not collect detailed information about relationships with family. However, using our best item (serious problems getting along with ‘other family member’ during the past 12 months), we found that although the childhood SLEs were significantly associated with this current family relationship variable ( P < 1 × 10 − 200 ), the PGS and childhood SLE associations remained significant when adjusting for this covariate.
In conclusion, among adults with more severe or recurrent forms of depression, higher PGSs for ADHD, depression, anxiety, and schizophrenia were consistently associated with higher reporting of exposure to SLEs. Notably however, our post-hoc analysis with non-psychiatric PGSs suggest a more complex story. While the psychiatric PGS were more consistently associated with childhood SLEs, and had stronger associations with each cumulative SLE variable, the magnitude and pattern of association for the past-year/lifetime SLEs were more similar among the psychiatric and non-psychiatric PGS (particularly for the T2D-PGS). Replication and follow-up causal analyses of these post-hoc results is warranted. Altogether, our results suggest that efforts to help genetically-vulnerable individuals cope with stress could potentially improve their outcomes and help break the cycle of recurrent depression and SLEs.
Data availability
Access to AGDS data is restricted due to the ethical guidelines governing the study but may be accessible following ethical review and data transfer agreements. Please contact Nicholas Martin ([email protected]) with queries related to accessing AGDS data.
Code availability
R analysis scripts used to run the statistical models can be shared upon reasonable request.
Herrman H, Patel V, Kieling C, Berk M, Buchweitz C, Cuijpers P, et al. Time for united action on depression: a Lancet-world Psychiatric Association Commission. Lancet . 2022;399:957-1022
Monroe SM, Simons AD. Diathesis-stress theories in the context of life stress research: implications for the depressive disorders. Psychol Bull. 1991;110:406–25.
Article CAS PubMed Google Scholar
Peyrot WJ, Milaneschi Y, Abdellaoui A, Sullivan PF, Hottenga JJ, Boomsma DI, et al. Effect of polygenic risk scores on depression in childhood trauma. Br J Psychiatry. 2014;205:113–9.
Article PubMed PubMed Central Google Scholar
Colodro-Conde L, Couvy-Duchesne B, Zhu G, Coventry WL, Byrne EM, Gordon S, et al. A direct test of the diathesis–stress model for depression. Mol Psychiatry. 2018;23:1590–6.
World Health Organization. World mental health report: transforming mental health for all. Geneva. 2022.
Paykel ES. Basic concepts of depression. Dialogues Clin Neurosci. 2008;10:279–89.
Warrier V, Kwong ASF, Luo M, Dalvie S, Croft J, Sallis HM, et al. Gene-environment correlations and causal effects of childhood maltreatment on physical and mental health: a genetically informed approach. Lancet Psychiatry. 2021;8:373–86.
Kendler KS, Karkowski LM, Prescott CA. The assessment of dependence in the study of stressful life events: validation using a twin design. Psychol Med. 1999;29:1455–60.
Bemmels HR, Burt SA, Legrand LN, Iacono WG, McGue M. The heritability of life events: an adolescent twin and adoption study. Twin Res Hum Genet. 2008;11:257–65.
Article PubMed Google Scholar
Kendler KS, Neale M, Kessler R, Heath A, Eaves L. A twin study of recent life events and difficulties. Arch Gen Psychiatry. 1993;50:789–96.
Plomin R, Lichtenstein P, Pedersen NL, McClearn GE, Nesselroade JR. Genetic influence on life events during the last half of the life span. Psychol Aging. 1990;5:25–30.
Kendler KS, Karkowski LM, Prescott CA. Causal relationship between stressful life events and the onset of major depression. Am J Psychiatry. 1999;156:837–41.
Kendler KS. Major depression and the environment: a psychiatric genetic perspective. Georg Thieme Verl. 1998;31:5–9.
CAS Google Scholar
Hammen C. Stress generation in depression: reflections on origins, research, and future directions. J Clin Psychol. 2006;62:1065–82.
Liu RT, Alloy LB. Stress generation in depression: a systematic review of the empirical literature and recommendations for future study. Clin Psychol Rev. 2010;30:582–93.
Kendler KS, Baker JH. Genetic influences on measures of the environment: a systematic review. Psychol Med. 2007;37:615–26.
Arnau-Soler A, Adams MJ, Clarke T-K, MacIntyre DJ, Milburn K, Navrady L, et al. A validation of the diathesis-stress model for depression in Generation Scotland. Transl Psychiatry. 2019;9:25.
Musliner KL, Andersen KK, Agerbo E, Albiñana C, Vilhjalmsson BJ, Rajagopal VM, et al. Polygenic liability, stressful life events and risk for secondary-treated depression in early life: a nationwide register-based case-cohort study. Psychol Med. 2021;1–10.
Peyrot WJ, Van der Auwera S, Milaneschi Y, Dolan CV, Madden PAF, Sullivan PF, et al. Does childhood trauma moderate polygenic risk for depression? A meta-analysis of 5765 subjects from the psychiatric genomics consortium. Biol psychiatry. 2018;84:138–47.
Mullins N, Power RA, Fisher HL, Hanscombe KB, Euesden J, Iniesta R, et al. Polygenic interactions with environmental adversity in the aetiology of major depressive disorder. Psychol Med. 2016;46:759–70.
Clarke TK, Zeng Y, Navrady L, Xia C, Haley C, Campbell A, et al. Genetic and environmental determinants of stressful life events and their overlap with depression and neuroticism. Wellcome Open Res. 2018;3:11.
Musliner KL, Seifuddin F, Judy JA, Pirooznia M, Goes FS, Zandi PP. Polygenic risk, stressful life events and depressive symptoms in older adults: a polygenic score analysis. Psychol Med. 2015;45:1709–20.
Ratanatharathorn A, Koenen KC, Chibnik LB, Weisskopf MG, Rich-Edwards JW, Roberts AL. Polygenic risk for autism, attention-deficit hyperactivity disorder, schizophrenia, major depressive disorder, and neuroticism is associated with the experience of childhood abuse. Mol Psychiatry. 2021;26:1696–705.
Peel AJ, Purves KL, Baldwin JR, Breen G, Coleman JRI, Pingault JB, et al. Genetic and early environmental predictors of adulthood self-reports of trauma. Br J Psychiatry. 2022;221:613–20
Hickie IB, Scott EM, Cross SP, Iorfino F, Davenport TA, Guastella AJ, et al. Right care, first time: a highly personalised and measurement-based care model to manage youth mental health. Med J Aust. 2019;211:S3–s46.
Hickie IB, Hermens DF, Naismith SL, Guastella AJ, Glozier N, Scott J, et al. Evaluating differential developmental trajectories to adolescent-onset mood and psychotic disorders. BMC Psychiatry. 2013;13:303.
Byrne EM, Kirk KM, Medland SE, McGrath JJ, Colodro-Conde L, Parker R, et al. Cohort profile: the Australian genetics of depression study. BMJ Open. 2020;10:e032580.
Elm EV, Altman DG, Egger M, Pocock SJ, Gøtzsche PC, Vandenbroucke JP. Strengthening the reporting of observational studies in epidemiology (STROBE) statement: guidelines for reporting observational studies. BMJ. 2007;335:806–8.
Article Google Scholar
Weathers FW, Blake DD, Schnurr PP, Kaloupek DG, Marx BP, Keane TM. The life events checklist for DSM-5 (LEC-5) – standard. 2013.
Brugha T, Bebbington P, Tennant C, Hurry J. The list of threatening experiences: a subset of 12 life event categories with considerable long-term contextual threat. Psychol Med. 1985;15:189–94.
Abecasis GR, Altshuler D, Auton A, Brooks LD, Durbin RM, Gibbs RA, et al. A map of human genome variation from population-scale sequencing. Nature. 2010;467:1061–73.
Purcell S, Neale B, Todd-Brown K, Thomas L, Ferreira MAR, Bender D, et al. PLINK: a tool set for whole-genome association and population-based linkage analyses. Am J Hum Genet. 2007;81:559–75.
Article CAS PubMed PubMed Central Google Scholar
Chang CC, Chow CC, Tellier LC, Vattikuti S, Purcell SM, Lee JJ. Second-generation PLINK: rising to the challenge of larger and richer datasets. Gigascience. 2015;4:7.
National Academies of Sciences E, and Medicine. Using population descriptors in genetics and genomics research: a new framework for an evolving field. Washington, DC. 2023.
Haplotype Reference Consortium. A reference panel of 64,976 haplotypes for genotype imputation. Nat Genet. 2016;48:1279–83.
Howard DM, Adams MJ, Clarke T-K, Hafferty JD, Gibson J, Shirali M, et al. Genome-wide meta-analysis of depression identifies 102 independent variants and highlights the importance of the prefrontal brain regions. Nat Neurosci. 2019;22:343–52.
Stahl EA, Breen G, Forstner AJ, McQuillin A, Ripke S, Trubetskoy V, et al. Genome-wide association study identifies 30 loci associated with bipolar disorder. Nat Genet. 2019;51:793–803.
Pardiñas AF, Holmans P, Pocklington AJ, Escott-Price V, Ripke S, Carrera N, et al. Common schizophrenia alleles are enriched in mutation-intolerant genes and in regions under strong background selection. Nat Genet. 2018;50:381–9.
Purves KL, Coleman JRI, Meier SM, Rayner C, Davis KAS, Cheesman R, et al. A major role for common genetic variation in anxiety disorders. Mol Psychiatry. 2020;25:3292–303.
Demontis D, Walters RK, Martin J, Mattheisen M, Als TD, Agerbo E, et al. Discovery of the first genome-wide significant risk loci for attention deficit/hyperactivity disorder. Nat Genet. 2019;51:63–75.
Nagel M, Jansen PR, Stringer S, Watanabe K, de Leeuw CA, Bryois J, et al. Meta-analysis of genome-wide association studies for neuroticism in 449,484 individuals identifies novel genetic loci and pathways. Nat Genet. 2018;50:920–7.
Lloyd-Jones LR, Zeng J, Sidorenko J, Yengo L, Moser G, Kemper KE, et al. Improved polygenic prediction by Bayesian multiple regression on summary statistics. Nat Commun. 2019;10:5086.
R Core Team. R: A Language and Environment for Statistical Computing. R Foundation for Statistical Computing: Vienna, Austria, 2022.
Thissen D, Steinberg L, Kuang D. Quick and easy implementation of the benjamini-hochberg procedure for controlling the false positive rate in multiple comparisons. J Educ Behav Stat. 2002;27:77–83.
Aas M, Bellivier F, Bettella F, Henry C, Gard S, Kahn JP, et al. Childhood maltreatment and polygenic risk in bipolar disorders. Bipolar Disord. 2020;22:174–81.
Park YM, Shekhtman T, Kelsoe JR. Interaction between adverse childhood experiences and polygenic risk in patients with bipolar disorder. Transl Psychiatry. 2020;10:326.
Kendler KS, Karkowski-Shuman L. Stressful life events and genetic liability to major depression: genetic control of exposure to the environment? Psychol Med. 1997;27:539–47.
Agnew-Blais JC, Wertz J, Arseneault L, Belsky DW, Danese A, Pingault JB, et al. Mother’s and children’s ADHD genetic risk, household chaos and children’s ADHD symptoms: a gene-environment correlation study. J Child Psychol Psychiatry. 2022;63:1153–63.
Hammen C, Shih JH, Brennan PA. Intergenerational transmission of depression: test of an interpersonal stress model in a community sample. Am Psychol Assoc. 2004;72:511–22.
Google Scholar
Sudre G, Frederick J, Sharp W, Ishii-Takahashi A, Mangalmurti A, Choudhury S, et al. Mapping associations between polygenic risks for childhood neuropsychiatric disorders, symptoms of attention deficit hyperactivity disorder, cognition, and the brain. Mol Psychiatry. 2020;25:2482–92.
Jansen PR, Polderman TJC, Bolhuis K, van der Ende J, Jaddoe VWV, Verhulst FC, et al. Polygenic scores for schizophrenia and educational attainment are associated with behavioural problems in early childhood in the general population. J Child Psychol Psychiatry. 2018;59:39–47.
Crouse JJ, Carpenter JS, Song YJC, Hockey SJ, Naismith SL, Grunstein RR, et al. Circadian rhythm sleep-wake disturbances and depression in young people: implications for prevention and early intervention. Lancet Psychiatry. 2021;8:813–23.
Jones HJ, Stergiakouli E, Tansey KE, Hubbard L, Heron J, Cannon M, et al. Phenotypic manifestation of genetic risk for schizophrenia during adolescence in the general population. JAMA Psychiatry. 2016;73:221–8.
Download references
Acknowledgements
We are indebted to all of the participants for giving their time to contribute to this study. We thank all the people who helped in the conception, implementation, beta testing, media campaign, and data cleaning. We thank the research participants and employees of 23andMe, inc., for making this work possible. The study protocol used by 23andMe was approved by an external AAHRPP-accredited institutional review board. The Australian Genetics of Depression Study was primarily funded by grant 1086683 from the National Medical Health and Research Council (NHMRC) of Australia. This work was further supported by an NHMRC EL1 Investigator Grant (GNT2008196) awarded to Jacob Crouse, an NHMRC L3 Investigator Grant (GNT2016346) awarded to Ian Hickie, and a philanthropic donation from families who affected by mental illness (who would like to be left anonymous).
Open Access funding enabled and organized by CAUL and its Member Institutions.
Author information
Authors and affiliations.
Brain and Mind Centre, Faculty of Medicine and Health, University of Sydney, Sydney, NSW, Australia
Jacob J. Crouse, Shin Ho Park, Jan Scott & Ian B. Hickie
Institute for Molecular Bioscience, The University of Queensland, Brisbane, QLD, Australia
Enda M. Byrne, Tian Lin & Naomi R. Wray
Child Health Research Centre, The University of Queensland, Brisbane, QLD, Australia
Enda M. Byrne
Mental Health and Neuroscience Program, QIMR Berghofer Medical Research Institute, Brisbane, QLD, Australia
Brittany L. Mitchell, Sarah E. Medland & Nicholas G. Martin
Academic Psychiatry, Institute of Neuroscience, Newcastle University, Newcastle, UK
Norwegian University of Science and Technology, Trondheim, Norway
Université de Paris, Paris, France
Queensland Brain Institute, The University of Queensland, Brisbane, QLD, Australia
Naomi R. Wray
Department of Psychiatry, University of Oxford, Oxford, UK
Oxford Big Data Institute, Li Ka Shing Centre for Health Information and Discovery, University of Oxford, Oxford, UK
You can also search for this author in PubMed Google Scholar
Contributions
JJC : Conceptualization; Formal analysis; Visualization; Writing – original draft; Writing – review & editing. SHP : Formal analysis; Visualization; Writing – review & editing. EMB : Data curation; Funding acquisition; Investigation; Methodology; Writing – review & editing. BLM : Data curation; Investigation; Methodology; Writing – review & editing. JS : Supervision; Writing – review & editing. SEM : Data curation; Funding acquisition; Investigation; Methodology; Writing – review & editing. TL : Formal analysis; Visualization; Writing – review & editing. NRW : Data curation; Funding acquisition; Investigation; Methodology; Writing – review & editing. NGM : Conceptualization; Data curation; Funding acquisition; Investigation; Methodology; Writing – review & editing. IBH : Conceptualization; Data curation; Funding acquisition; Investigation; Supervision; Writing – review & editing
Corresponding author
Correspondence to Ian B. Hickie .
Ethics declarations
Competing interests.
Ian B. Hickie is the Co-Director, Health and Policy at the Brain and Mind Centre (BMC) University of Sydney, Australia. The BMC operates an early-intervention youth services at Camperdown under contract to headspace. He has previously led community-based and pharmaceutical industry-supported (Wyeth, Eli Lily, Servier, Pfizer, AstraZeneca) projects focused on the identification and better management of anxiety and depression. He is the Chief Scientific Advisor to, and a 3.2% equity shareholder in, InnoWell Pty Ltd. InnoWell was formed by the University of Sydney (45% equity) and PwC (Australia; 45% equity) to deliver the $30 M Australian Government-funded Project Synergy (2017-20) and to lead transformation of mental health services internationally through the use of innovative technologies.
Additional information
Publisher’s note Springer Nature remains neutral with regard to jurisdictional claims in published maps and institutional affiliations.
Supplementary information
Supplementary figures, supplementary tables, rights and permissions.
Open Access This article is licensed under a Creative Commons Attribution 4.0 International License, which permits use, sharing, adaptation, distribution and reproduction in any medium or format, as long as you give appropriate credit to the original author(s) and the source, provide a link to the Creative Commons licence, and indicate if changes were made. The images or other third party material in this article are included in the article’s Creative Commons licence, unless indicated otherwise in a credit line to the material. If material is not included in the article’s Creative Commons licence and your intended use is not permitted by statutory regulation or exceeds the permitted use, you will need to obtain permission directly from the copyright holder. To view a copy of this licence, visit http://creativecommons.org/licenses/by/4.0/ .
Reprints and permissions
About this article
Cite this article.
Crouse, J.J., Park, S.H., Byrne, E.M. et al. Patterns of stressful life events and polygenic scores for five mental disorders and neuroticism among adults with depression. Mol Psychiatry (2024). https://doi.org/10.1038/s41380-024-02492-x
Download citation
Received : 06 June 2023
Revised : 08 February 2024
Accepted : 20 February 2024
Published : 04 April 2024
DOI : https://doi.org/10.1038/s41380-024-02492-x
Share this article
Anyone you share the following link with will be able to read this content:
Sorry, a shareable link is not currently available for this article.
Provided by the Springer Nature SharedIt content-sharing initiative
Quick links
- Explore articles by subject
- Guide to authors
- Editorial policies

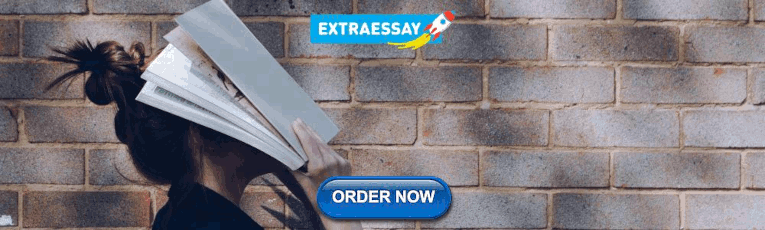
IMAGES
VIDEO
COMMENTS
The neurogenesis hypothesis of depression posits (1) that neurogenesis in the subgranular zone of the dentate gyrus is regulated negatively by stressful experiences and positively by treatment with antidepressant drugs and (2) that alterations in the rate of neurogenesis play a fundamental role in the pathology and treatment of major depression ...
Adult neurogenesis is the process by which functional, mature neurons are produced from neural stem cells (NSCs) in the adult brain. In most mammals, including humans, it only occurs in the subgranular zone of the hippocampus, and in the olfactory bulb. [1] The neurogenesis hypothesis of depression proposes that major depressive disorder is ...
In non-human primates, stress - a predisposing factor to depression in humans - decreased neurogenesis, and neurogenesis levels were normalized by antidepressants . These and many other studies gave the neurogenic hypothesis of depression its initial robust trajectory ( 2 ): adult-generated hippocampal neurons are needed for proper mood ...
Abstract. Newborn neurons are continuously added to the adult hippocampus. Early studies found that adult neurogenesis is impaired in models of depression and anxiety and accelerated by antidepressant treatment. This led to the theory that depression results from impaired adult neurogenesis and restoration of adult neurogenesis leads to recovery.
The neurogenesis hypothesis of depression posits (1) that neurogenesis in the subgranular zone of the dentate gyrus is regulated negatively by stressful experiences and positively by treatment ...
Understanding depression pathophysiology is challenging because varying depression symptomatology cannot be explained by single hypothesis. • Pathophysiologic mechanisms include: monoamine hypothesis, genetic, environmental, immunologic, endocrine factors and neurogenesis.
Depression. The past decade and a half of research on the role of adult neurogenesis in mental disorders has focused mainly on depression. The neurogenesis hypothesis of depression (Duman et al ...
The neurogenesis hypothesis of affective and anxiety disorders: are we mistaking the scaffolding for the building? Neuropharmacology. 2012;62:21-34. Article CAS PubMed Google Scholar
Neurogenesis is a process, not a time point , a message emphasized in the road sign (inset) that condenses the journey from aNSC to GCL neuron. (D and E) The two branches of the neurogenic hypothesis of depression. (D) The first branch proposes ablation of adult neurogenesis does not greatly influence mood under normal, nonstressful conditions.
The 'neurogenesis hypothesis of depression' emphasizes the importance of upregulated hippocampal neurogenesis for the efficacy of antidepressant treatment. Neuromodulation is a promising therapeutic method that stimulates neural circuitries to treat neuropsychiatric illnesses. We conducted a scoping review on the neurogenic and antidepressant ...
The 'neurogenesis hypothesis of depression' emphasizes the importance of upregulated hippocampal neurogenesis for the efficacy of antidepressant treatment. Neuromodulation is a promising therapeutic method that stimulates neural circuitries to treat neuropsychiatric illnesses. We conducted a scoping review on the neurogenic and ...
Based on current evidence, the neurogenic hypothesis for depression 94 warrants revision in that adult hippocampal neurogenesis is more likely a substrate for the behavioral effects of ...
The monoamine hypothesis posits that depression is caused by the alteration of monoamine levels, including serotonin (5HT), norepinephrine (NE), and dopamine (DA). A preliminary finding was that monoamine depletion by the antihypertensive drug reserpine caused depression in patients not previously affected by the disease.
Adult hippocampal neurogenesis has been hypothesized to play a potentially important role on the pathology and successful treatment of depression. A neurogenic hypothesis of depression has been postulated, which suggests that reduced adult hippocampal neurogenesis may underlie the pathoetiology of depression, while antidepressant efficacy ...
Abstract. Newborn neurons are continuously added to the adult hippocampus. Early studies found that adult neurogenesis is impaired in models of depression and anxiety and accelerated by antidepressant treatment. This led to the theory that depression results from impaired adult neurogenesis and restoration of adult neurogenesis leads to recovery.
Statement of hypothesis. As mentioned above, in a variety of species, stress is one of the important controlling influences on neurogenesis. Stress is also believed to be the most significant ...
The mechanisms underlying MDD are not understood. The neurogenic theory of depression suggests that impaired adult neurogenesis (AN) in the dentate gyrus (DG) triggers depression and that restoration of AN leads to recovery . AN refers to the process that generates new neurons beyond development in adulthood.
The neurogenesis theory of depression explains that the delay in mood improvement is a result of the minimal effect serotonin has on neurogenesis. Researchers have turned their focus to chemicals in the brain that promote neurogenesis and suggest that new treatments targeting said chemicals could be a more logical and effective treatment for ...
A Neurogenic Theory of Depression. Stress, an environmental factor, is an important causal factor in precipitating episodes of depression in human, and potently suppresses hippocampal neurogenesis in adult monkey (Gould et al. 1998; Malberg and Duman, 2003), probably due to increased glucocorticoid release (Gould et al. 1991; Cameron and Gould, 1994).
This hypothesis proposes that depression results from decreased neurotrophic support, leading to neuronal atrophy, decreased hippocampal neurogenesis and loss of glia, and that antidepressant treatment blocks or reverses this neurotrophic factor deficit, and thereby reverses the atrophy and cell loss [6,13].
These and other data have led us to propose the following theory regard-ing clinical depression. Stress-induced decreases in dentate gyrus neurogenesis are an ... Serotonin, neurogenesis and ...
The hypothesis that dysfunction of these regions contribute to depression development is consistent with evidence that lesions involving either the PFC or the striatum (a major target of efferent ...
These findings reveal alterations at the levels of intracellular signaling, gene expression, neurotrophic factors, neurogenesis, neuroinflammation, excitatory and inhibitory neurotransmission, and synaptic number and function, and have been described in several brain regions implicated in depression 13-22. The signaling pathways and types of ...
The dominant ('general') version of the diathesis-stress theory of depression views stressors and genetic vulnerability as independent risks. In the Australian Genetics of Depression Study (N ...