The role of a cosmetologist in the area of health promotion and health education: A systematic review
Affiliation.
- 1 Institute of Medical Sciences, Collegium Medicum, Jan Kochanowski University, Kielce, Poland.
- PMID: 33312929
- PMCID: PMC7723003
- DOI: 10.34172/hpp.2020.52
Background: Contemporary cosmetology, apart from beautifying and caring for the human body, deals also with prevention aimed at maintaining health and physical fitness as long as possible. The profession of a cosmetologist so understood is closely related to the modern concept of health promotion, the part of which is health education. The objective of this review was to evaluate whether a cosmetologist may be a health promoter, and whether a beauty salon mayserve as a place for conducting educational programs. Methods: A systematic review was done using several electronic databases such as PubMed(including MEDLINE), Web of Science Core Collection, Scopus, Embase, and Academic Search Ultimate (EBSCO) and related keywords. The studies published in English between 2008 and 2018 which had specifically mentioned the role of a cosmetologist in the area of health promotion and health education were included. Results: In total, 7 articles met the study criteria. It was found that cosmetologists have the potential to promote pro-health activities. The results of this review also suggest that beauty salons are suitable places for increasing pro-health awareness and can be successfully used to conduct educational programs about healthy lifestyle, as well as skin, breast and cervical cancer prevention. Conclusion: A well-educated and aware of health risks cosmetologist seems to be the right person to transmit and spread knowledge about the proper lifestyle in her workplace and the local environment. A beauty salon, as a place of social interaction, may constitute an area of implementation of pro-health educational programs.
Keywords: Beauty salons; Health education; Health promotion; Prevention; Women.
© 2020 The Author(s).
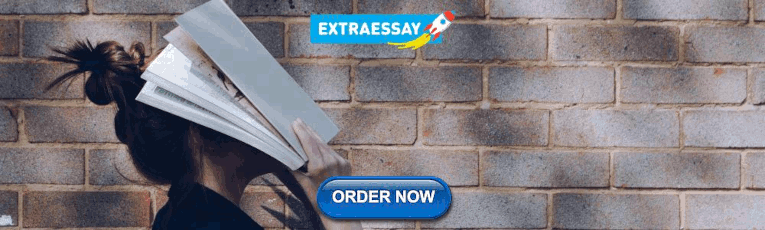
Publication types
Thank you for visiting nature.com. You are using a browser version with limited support for CSS. To obtain the best experience, we recommend you use a more up to date browser (or turn off compatibility mode in Internet Explorer). In the meantime, to ensure continued support, we are displaying the site without styles and JavaScript.
- View all journals
- ADVERTISEMENT FEATURE Advertiser retains sole responsibility for the content of this article
Recognizing the beauty of science, and the science behind beauty
Produced by
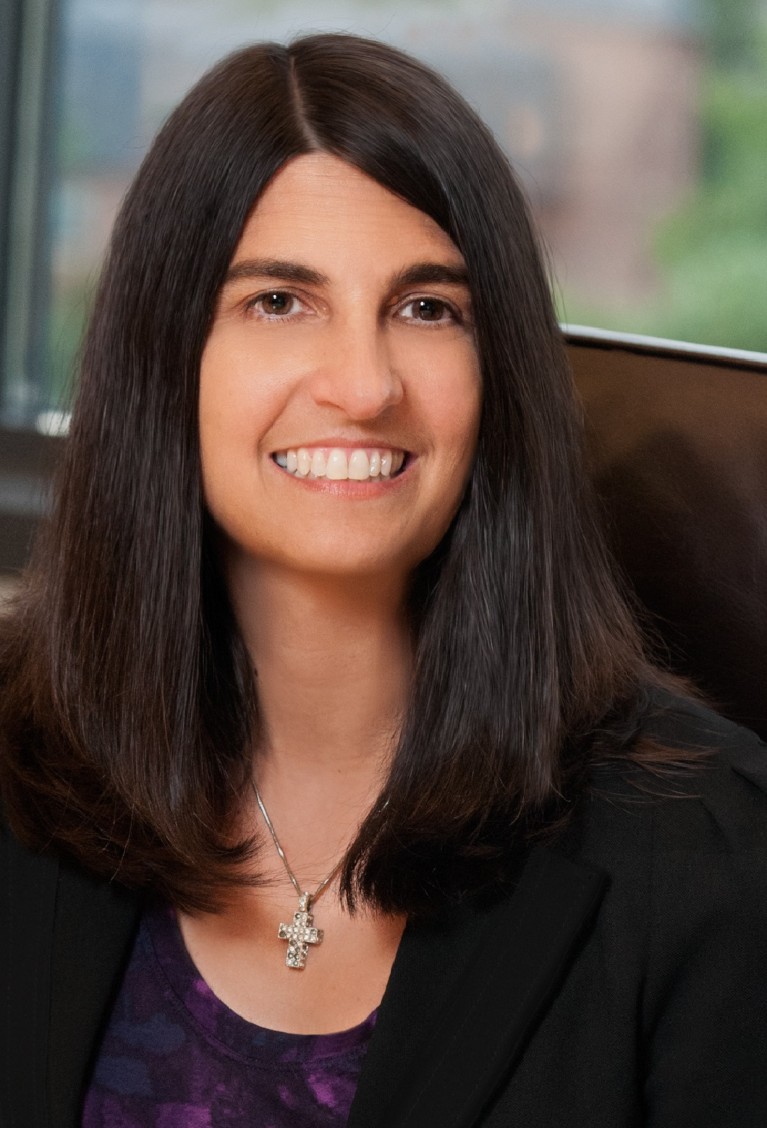
Lisa Napolione, Senior Vice President, Global Research & Development at The Estée Lauder Companies
The Estée Lauder Companies has a long history of science and innovation. Fifty years ago, the prestige beauty company created the world’s first allergy-tested, fragrance free skincare line — and it has continued to roll out transformative beauty products ever since. The Company recently partnered with Nature Research to create two new prizes designed to inspire women in science, technology, engineering or mathematics (STEM), one to honour early career female scientists making pioneering discoveries, and the other to recognize leaders — women and men — behind initiatives supporting greater equality in STEM. Biochemical engineer Lisa Napolione, who leads the Company’s R&D efforts, explains the impetus for the awards and how The Estée Lauder Companies takes a science-driven approach to skincare and beauty.
The new prizes are designed to inspire women in STEM, and focus on different things. Why put the spotlight on educators and young researchers?
Both of these areas are critical in their own right and integral to everything we do at The Estée Lauder Companies. We are a company that was founded by a pioneering woman who supported other women and who remains an inspiration to all of us — and so honouring exceptional female researchers through the Inspiring Science Award really spoke to us. We hope it not only shines a much-deserved light on the achievements of exceptional women in STEM, but also helps to establish a new generation of role models. The second award — the Innovating Science Award — recognizes a person or an organization that promotes STEM to girls and young women. I really feel strongly that young girls need role models and mentors in STEM, because without these influences we wouldn’t have the deep bench of research expertise among the next generation of scientists.
This all sounds very personal to you.
It is! I was so fortunate that early in my education, I had a mentoring role-model, Nora Kyser, who was one of the first female chemical and ceramic engineers in all of the United States. She was my high school chemistry teacher in my little hometown in western New York, and she arranged with the school district that, if she paid for her own research, she could work after hours in the school’s laboratory. She saw something in me, and hired me as her lab assistant. Her hands-on personal attention affected me so much. It was an amazing experience for which I will be forever grateful — and it inspired me to do for others what she did for me. I do what I do today because of her.
How does science inform how products are developed at The Estée Lauder Companies?
Many people in the scientific community don’t appreciate the breadth and depth of the serious science that happens in beauty. The Estée Lauder Companies’ R&D teams are constantly looking at breakthroughs in other fields of science and technology to inspire our skincare research. We conduct epigenetic research, including into sirtuins, nrf2 activation and cellular repair. Notably, we have conducted research on Nobel Prize-winning topics: Estée Lauder was the first cosmetic brand to research skin cells’ circadian rhythm and the role of ‘clock genes’ n cellular repair, and the first to research autophagy and its role in helping repair skin-cell damage. Last year, Estée Lauder R&D started mechanobiology research to help understand why and how blinking ages the look of the eyes, and now how light pollution, specifically blue light at night, desynchronizes skin cells’ natural repair.
Much of our research uses nature as the ultimate inspiration with more than 12,000 bioactive molecules in our database supported by hundreds of technical measures and assays. Plus, we have fermentation and biotechnology capabilities that allow us to produce high-performance ingredients in a multitude of organisms, including bacteria, yeast and mould. The power of fermentation can be seen in our La Mer brand, which is built around the Miracle Broth™ created from a unique three to four-month fermentation with a specific sea kelp. In all, this research has resulted in new products that can help protect against the visible effects of pollution, improve skin tone, reduce undesired pigmentation, and counteract visible skin and hair ageing. We are always looking at relevant applications in emerging scientific areas that have yet to fully materialize — because if it’s hot in science, it will be hot in beauty.
What’s an example of this process in action?
We took a multi-pronged approach for the Clinique Even Better Clinical product for improving skin tone and visibly correcting dark spots. It’s such an interesting area of science, because there are multiple pathways behind the abnormal accumulation of melanin, the pigment responsible for these discolouration issues. We explored several approaches: new antioxidant ingredients that protect against free radicals; biofermentation technologies that target melanin clusters; and exfoliating agents to get rid of the dead skin cells that contain melanin dust. This all required a very detailed understanding of the underlying biology and a degree of scientific rigour that’s absolutely necessary for us to deliver products that really work.
Quick links
- Explore articles by subject
- Guide to authors
- Editorial policies
Advertisement
Biocosmetics: technological advances and future outlook
- Challenges in Managing and Risk Assessment of Emerging Environmental Pollutants
- Published: 25 November 2021
- Volume 30 , pages 25148–25169, ( 2023 )
Cite this article
- Nishu Goyal ORCID: orcid.org/0000-0003-4371-7012 1 &
- Frankline Jerold 1
34k Accesses
36 Citations
4 Altmetric
Explore all metrics
The paper provides an overview of biocosmetics, which has tremendous potential for growth and is attracting huge business opportunities. It emphasizes the immediate need to replace conventional fossil-based ingredients in cosmetics with natural, safe, and effective ingredients. It assembles recent technologies viable in the production/extraction of the bioactive ingredient, product development, and formulation processes, its rapid and smooth delivery to the target site, and fosters bio-based cosmetic packaging. It further explores industries that can be a trailblazer in supplying raw material for extraction of bio-based ingredients for cosmetics, creating biodegradable packaging, or weaving innovation in fashion clothing. Lastly, the paper discusses what it takes to become the first generation of a circular economy and supports the implementation of strict regulatory guidelines for any cosmetic sold globally.
Similar content being viewed by others
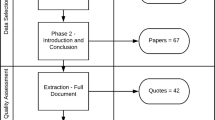
Concepts and forms of greenwashing: a systematic review
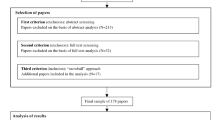
Sustainability trends and gaps in the textile, apparel and fashion industries
Thermal Processing and Canning
Avoid common mistakes on your manuscript.
Introduction
Biocosmetics are cosmetic products made from 100% natural ingredients derived from plants, animals, microbes, enzymes, insects, and organic crops that are free of pesticides and chemical fertilizers and used for topical skin, hair, face, and oral care (Vandamme 2001 ; Novak et al. 2014 ). Most of the conventional skin-care cosmetic formulations use petroleum or mineral oil-derived ingredients, which are harmful and non-biodegradable. To achieve a circular economy while satisfying customer demand for green cosmetics and addressing environmental concerns, many cosmetic giants have diverted their attention from fossil-based ingredients to bio-based ingredients. The current market for sustainable, natural, and greener cosmetics is massive because such products garner trust and respect by fairly treating nature. Additionally, government support and faster product approval for biocosmetics are creating a favorable climate for big business players such as The Estée Lauder Companies, Inc. (USA), LOréal SA (France), Bare Escentuals, Inc. (USA), Nature’s Gate (USA), Aubrey Organics, Inc. (USA), and FANCL Corp. (Japan). This market has seen a sharp rise over the period 2018–2021 (Market Research Report 2019 ). As per market analysis reports related to skincare products, the global organic and natural cosmetic market is expected to reach USD25.11 billion by 2024 (Market Research Report 2021 ). Biocosmetic products with standard organic certification labels such as Ecocert, Cosmébio, NaTrue, USDA Organic, BDIH, and Soil Association allow their commercialization worldwide. The distribution channel for such products includes drugstores, pharmacies, organic food shops, health food retailers, department stores, beauty retailers, and online shopping sites.
This review highlights the importance of key ingredients in a cosmetic formulation and assesses the environmental and health risks associated with conventional petroleum-based ingredients. Active ingredients extracted from renewable bio-based alternatives are preferred because they are nontoxic and biodegradable. The search for high-performing, safe, and sustainable ingredients led many cosmetic manufacturers worldwide to explore the processes for producing natural and greener cosmetics. This review unifies technological innovation and industry in sourcing/extracting bio-based ingredients using lab cultivation processes, green synthesis of nanoformulations for fast and effective delivery, and selecting the best bioactive ingredient during process formulation steps using computational modeling. These scientific achievements are highly promising in incorporating new generation safe ingredients into our cosmetic products and in achieving zero waste goals.
The chemistry of a cosmetic formulation
The chemical composition of the ingredients and their doses in the formulation decides the potential impact of the formulation on our health. A typical cosmetic contains about 15–20 ingredients and considering, the use of at least five cosmetic products per day; we are placing 75–100 chemicals on our skin through cosmetic use (Jones and Selinger 2017 ). While the formula of a product may differ, the key ingredients in a cosmetic formulation are water, emulsifier, preservatives, thickeners, color, emollient, and fragrances (Darling 1959 ). Ultra-pure distilled water is the basis of almost every formulation, including creams, lotions, makeup, deodorants, shampoos, and conditioner, and acts as a solvent to emulsify for consistency. Emulsifiers such as potassium cetyl sulfate and polysorbates are added to relieve the surface tension between oil and water and form a homogenous and uniform texture of the formulation. A water-soluble preservative such as paraben, formaldehyde, salicylic acid, and benzyl alcohol is added to extend the shelf life of the product and prevent the growth of microorganisms. The concentration of preservatives may range from 0.01 to 5% (Benson et al. 2019 ). Thickeners, as the name suggests, increase the viscosity of the formulation by absorbing water and oil. Thickeners can be synthetic, natural, mineral, or lipid-based. Coloring agents/pigments are added to appeal to the users and fulfill their demands. A huge range of substances is used in this category including, both organic and inorganic. Organic substances come from plants or animals, such as beet powder, cochineal extract, while inorganic substances include coal tar, chromium oxide, and manganese. Emollients such as beeswax, coconut oil, olive oil, petroleum jelly, mango butter, and glycerine are used to soften the skin by reducing water loss. Fragrances are added to add a pleasant smell, which is a key factor in consumer decisions and is considered a trade secret. The USA alone approved approx. 12,500 unique chemical ingredients for personal care products (Oh and Kim 2020 ; Wang et al. 2020 ).
The transition from fossil fuel-based to bio-based cosmetics
The use of chemicals in cosmetic formulations has raised several questions on its safety aspects. Several reports in social media or news websites relate to potentially toxic compounds viz. lead, aluminum, mercury, parabens, etc., present in a cosmetic formulation. Panico et al. 2019 (Panico et al. 2019 ) conducted a detailed study to assess the presence of potentially toxic substances by examining product labels. A total of 283 products, collected from various shops in Italy, were divided into three categories: rinse-off (toothpaste, shower gel, liquid soap, shampoo, soap, and shaving foam), leave-on (moisturizer, face/hand cream, sunscreen, and aftershave deodorant), and make-up (lip balm, lipstick, nail polish, and foundation). A total of 26 compounds were identified in the fragrances category as skin sensitizers, skin irritants, and allergens. Benzophenone, a component of sunscreen, is carcinogenic to humans, and due to its good lipophilic properties, this substance is absorbed in high concentrations and can be detected in biological fluids including mother’s milk (Author 2010 ). Toluene and dibutyl phthalate, used in several cosmetic products and nail polish, enter through both dermal and inhalation routes and cause reproductive toxicity and damage to the nervous system (Hyun and Byung 2004 ; Kopelovich et al. 2015 ). A review by Matwiejczuk et al. 2020 (Matwiejczuk et al. 2020 ) on the use of parabens and their derivatives as preservatives in cosmetic applications stated the presence of parabens in the body in a non-metabolized form which enters by absorption, transformation, and accumulation. Few studies on cultured skin cells proved the undesirable effect of paraben on keratinocytes, fibroblast, and collagen expression (Darbre and Harvey 2008 ). There are dozens of studies on the safety of parabens, and hence many countries banned the use of this chemical in personal care products for infants and children (Vinet and Zhedanov 2011 ). Prolonged exposure to triclosan, a cosmetic preservative, can cause liver fibrosis, disrupt hormonal balance, impair muscle contraction and reduce bacterial resistance and its recommendable concentrate is up to 0.3% (Dinwiddie et al. 2014 ; Dhillon et al. 2015 ; Lee et al. 2019 ). Above this triclosan concentration, the word “poison” has to be labeled. Trace presence of heavy metals such as lead (Pb), aluminum (Al), chromium (Cr), cadmium (Cd), zinc (Zn), titanium (Ti), manganese (Mn) in lip products have always been a concern and invites safety regulations (Al-Saleh et al. 2009 ; Brown 2013 ; R. Sahu, P. Saxena 2014 ; SCCS and Rousselle 2015 ; Kaličanin and Velimirović 2016 ). Limonene, widely used for fragrance in cosmetic products, is a potential allergen (Nardelli et al. 2011 ; Bråred Christensson et al. 2016 ). Given these estimates, we could potentially be exposing ourselves to a variety of unknown hazardous chemicals, and hence a systematic and intelligent move to replace chemical cosmetics with biological cosmetics or preferring alternative substances of natural origin is of considerable interest. Table 1 lists chemical ingredients of concern used in cosmetic formulations that need immediate replacement with ingredients of natural origin.
Concerned with the toxicity of ingredients, the Australian Government’s National Industrial Chemicals Notification and Assessment Scheme (NICNAS) set a regulatory control on the import, use, and manufacturing of cosmetic chemicals in Australia so that every ingredient contained in the product is scientifically assessed to ensure the safety of the user (Phua 2020 ). The European Union (EU) banned 1328 chemicals used in cosmetics that are responsible for a genetic disorder, birth defect, and reproductive harms, while the number of ingredients banned by the Food and Drug Administration, USA is a mere 12, possibly due to lack of updates in federal regulations since 1938 or strong favoritism toward cosmetic manufacturing companies (Alexa Riccolo 2021 ; Pistollato et al. 2021 ). Other countries such as Canada, Japan, China, Ethiopia, India, Cambodia, and Vietnam are also banning the chemical ingredients or amending the existing regulations to satisfy the public safety concerns and spreading awareness through warnings on cosmetic labels (Raj and Chandrul 2016 ; Pistollato et al. 2021 ).
In addition to the toxicity of fossil-based ingredients, several other factors such as environment, sustainability, cost, effectiveness, and long-term health push for the much-needed transition from conventional to bio-based cosmetics. Due to a rapid decline in nonrenewable sources and strict environmental regulation, the market demand for natural products derived from oils, agricultural plants, and bacteria has increased substantially over the past few years (De Mejia et al. 2020). Bio-based personal care products offer waste reduction, recycling, low-energy consumption, safety, and well-being (Secchi et al. 2016 ). Natural and oleochemical ingredients such as 2-ethyl hexyl stearate, cetyl stearyl, and polyhexanide render natural healing, increased effectiveness, and fewer allergic reactions (Kulka 2009 ). They are safer and cheaper to produce, source, and ship compared to their chemical alternatives. Their low cost is due to the abundance of raw materials in Southeast Asia with modern biorefinery technologies and safety owing to the bio-based processes such as fermentation that are not only safe but also less energy-intensive. An example is butylene glycol, a widely used emollient and humectant in cosmetics, produced by fermentation of plant-based sugars making it a more natural and sustainable alternative than catalytic dehydrogenation of carcinogenic acetaldehyde (Fulmer et al. 1933 ; Dwivedi 2020 ). Conscious consumers and manufacturers care for social and environmental responsibilities. However, a lack of knowledge and standardization for “eco-labels” allow manufacturers, suppliers, and advertisers to use this term voluntarily without any compulsion or consequences (Cervellon and Carey 2012 ). Selling natural ingredients in cosmetics is also a secret marketing strategy for industries to enhance their brand value and improve customer loyalty.
Active ingredients and adjuvants
The active ingredient is a term for a pharmaceutical drug that is biologically active. In cosmetics, the active ingredient is a marketing terminology and refers to substances claimed in skincare products to target skin concerns. The skin has three main layers i.e., epidermis, dermis, and subcutaneous tissue, and a few appendages such as hair, nail, sweat, and sebaceous gland. As shown in Fig. 1 , the route of transport of a cosmetic ingredient through the skin can be transepidermal (intercellular or transcellular) and transappendageal. The delivery through the latter is of low importance because it constitutes a very small area (01–1%) of the skin surface. Skin influences penetration of active ingredients depending on physiological factors such as skin permeation and skin retention (dependent on age, ethnicity, gender, and skin disorder), drug physicochemical properties, and the properties of the vehicle (Soares et al. 2015 ).
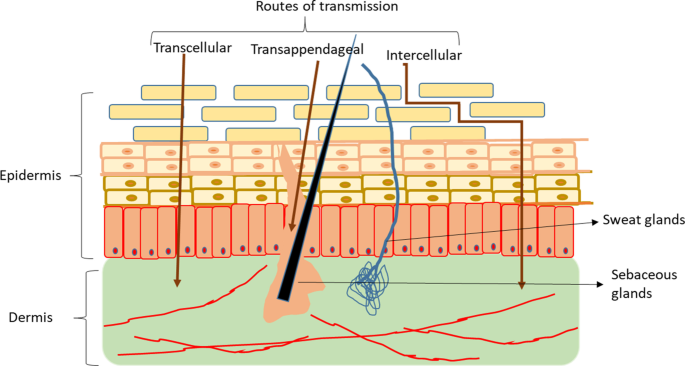
Various routes of ingredients delivery through the skin
Active ingredients are often supported with scientific studies to show the efficacy of the target and categorized into antioxidants, astringents, exfoliant, humectant, skin conditioner, and surfactant. Examples are, alpha/beta hydroxy acids (AHA/BHA), ceramides, hyaluronic acid (HA), niacinamide, peptides, retinol, vitamin C, E, etc. (Darling 1959 ). AHA/BHA works by exfoliating the dead skin by dissolving the bonds between skin cells. Ceramides are fat molecules that protect our skin from pollution and other environmental stressors and keep the skin hydrated and moisturized. HA, a moisture-binding ingredient, keep the skin soft and radiant and is mostly used in anti-aging skincare products. Niacinamide has anti-inflammatory properties and boosts the level of fatty acid in our skin. Peptides signal the body to produce more collagen, retinol is a derivative of vitamin A, which promotes skin renewal, boosts cell turnover, and is used for acne-fighting ability. Vitamin C is proven as an antioxidant scientifically to boost the synthesis of collagen, minimize scars and wrinkles. Alpha-lipoic acid is an antioxidant and is soluble in both oil and water.
Adjuvants are ingredients that help in preparing the formulation with desired properties to ensure delivery of the active ingredients, for example, emulsifier, buffer, thickener, preservative, surfactant, fragrance, emollient, humectant, occlusive, and penetration enhancer. They determine the product form, such as gel, serum, cream, etc.; maintain the product texture, enhance the performance of active ingredients, prevent microbial growth and chemical decomposition, and help to make the product smell nice and look good. The label on a cosmetic product lists the ingredients used for its preparation as per International Nomenclature of Cosmetic Ingredients (INCI) names. The ingredients are listed in decreasing order of their content in the formulation. This information gives the customer an informed choice for choosing a product.
Ingredients used in biocosmetics, active, or adjuvants, can be categorized as animal or vegetable-derived fats, waxes and oils, essential oils and oleoresins, vegetable saps and extracts, raw plant materials, and coloring matter of animal, or vegetable origin. Europe, the largest cosmetic market in the world, provides enough opportunities to developing countries to export natural ingredients (Centre for the Promotion of Imports from developing countries 2020 ). Due to increasing consumer demand and the need for sustainable raw materials, companies are putting efforts to replace synthetic ingredients with their natural variants. Table 2 lists common feedstock imported by European countries for bio-based chemicals (Centre for the Promotion of Imports from developing countries 2020 ). The top six natural ingredients in the European cosmetic market are shea butter, coconut oil, mango butter, frankincense essential oil, patchouli essential oil, and licorice extract. Since 1992, Greentech group, a France-based biocosmetic firm and one of the importers in the list has been using plants, algae, micro-algae, and microbes to extract/develop active ingredients. URBALYS®, obtained from Shisandra Chinensi , EXPOZEN®, obtained from Halymenia durvillei , SOLIBERINE® obtained from Buddleja officials flowers, and HEBELYS®, obtained from optimized fermentation of Sphingomonas sp. are some of the newly launched products by Greentech group using new generation skincare active ingredients (Filaire 2019 ).
Technological advances favoring biocosmetics
Green synthesis of nanoparticles.
Nanotechnology in cosmetics plays a big role in better performance, non-cytotoxicity, and bioavailability of active ingredients in personal care products. The active ingredients adsorb on the surface of nanoparticles and help in efficient absorption, increased color and finish quality, and enhanced penetration through the skin. Modifying the shape, chemical composition, size, solubility, and chemical reactivity of the nanoparticles used in the cosmetic formulation helps in further enhancement of cosmetic products such as shelf life, efficacy, and performance (Chaudhri et al. 2015 ). Different metal nanoparticles are used in cosmetic formulations, such as silver (Ag), gold (Au), titanium (Ti), zinc oxide (ZnO), Mica, platinum, alumina, copper, and fullerenes (Chiari-Andréo et al. 2019 ). TiO2 and ZnO nanoparticles are nonoily and can be easily absorbed. TiO2, ZnO, and ZrO2 nanoparticles act as UV filters and are used in sunscreens, lip balms, and moisturizers (Wawrzynczak et al. 2016 ). Several research papers outline the pros and cons of nanotechnology in cosmetic formulations and describe their various types which impart different characteristics to cosmetic products (Mihranyan et al. 2012 ; Raj et al. 2012 ) (Ekpa Effiong et al. 2020 ). Nanoparticles used in cosmetics are liposomes, nanoemulsions, nanocapsules, solid lipid nanoparticles, nanocrystals, nanosilver, nanogold, dendrimer, hydrogel, etc. The synthesis and characterization procedure is also well described in the literature (Paiva-Santos et al. 2021 ) (Naveed Ul Haq et al. 2017 )(Harishchandra et al. 2020 ). However, due to the small size of these nanoparticles, they can easily enter the bloodstream through skin or via inhalation to be transported to various organs. Malfunction of vital organs is also possible with higher doses and longer residence time of nanoparticles (Oberdörster et al. 2005 ; Subhashini et al. 2017 ). Environmental concerns have also been raised due to the release of nanoparticles into the air, water, and soil during their manufacture, use, and disposal. The ability of zinc oxide (ZnO), silver (Ag), titanium dioxide (TiO2), cerium oxide (CeO2), Cu, and Fe metal nanoparticles to induce oxidative stress has been reported (Manke et al. 2013 ). Fullerenes are known to kill water fleas besides bacteriocidal properties and the potential of biomagnification (Brunet et al. 2009 ). The toxicity and regulatory aspect of nanotechnology-based products need careful consideration before implementing them in direct contact with our skins.
Due to drawbacks associated with conventional methods of synthesizing metal-based nanoparticles, green synthesis of nanoparticles is receiving attention due to nontoxic, clean, and eco-friendly methods which protect the ecology and restore the quality of the environment (Paiva-Santos et al. 2021 ) (Arroyo et al. 2020 ) (Keijok et al. 2019 ). Green synthesis of silver nanoparticles hybrid from the natural extract by Arroyo et al. 2019 (Arroyo et al. 2020 ) was found to be highly effective and an affordable alternative for the market. Abdullah et al. 2021 (Tan Sian Hui Abdullah et al. 2021 ) reported the synthesis, characterization, and application of Ag-nanoparticles from the extract of red-onion peel and display higher antioxidant properties as compared to ascorbic acid. These studies suggest the development of nonhazardous and biocompatible green catalysts for organic transformations. Biocompatibility and safety aspects of Phyto-metal nanoparticles (Phyto-MNPs), plant-mediated green synthesis of nanoparticles, synthesized for dermo-pharmaceutical, and cosmetic applications is detailed in a review by Paiva-Sentos et al. 2021 and suggests the high potential of green synthesis toward clinical translation.
Biotechnological ingredients: safe and effective
The contribution of biotechnological processes toward the cosmetic market is huge owing to the production of safe and effective active ingredients via low-cost and contamination-free methods. Gomes et al. 2020 (Gomes et al. 2020 ) discuss some of these biotechnological processes and products used for developing cosmetic formulations. Some of the active ingredients resulting from biotechnological processes are kojic acid (Lajis et al. 2013 ), hyaluronic acid (Pan et al. 2015 ; Cheng et al. 2017 ), resveratrol (Donnez et al. 2009 ), human epidermal growth factors (Ferrer-Tasies et al. 2021 ), superoxide dismutase (Carroll et al. 2007 ), and photolyases (Marizcurrena et al. 2020 ). Traditional biotechnology exploits the potential of microbes via fermentation, while modern biotechnology manipulates the genetic material and inserts it in a suitable host for the desired application. During fermentation, microbes/enzymes are used as biocatalysts in bioreactors for large-scale production of proteins, secondary metabolites, and cosmetic ingredients, and the process is further optimized for enhanced productivity and yield (Sajna et al. 2015 ). Fermented food items viz. sauerkraut, kimchi, cheese, tempeh, beer or wine, etc. are well-accepted and possess multiple benefits. Similarly, fermented ingredients for cosmetics such as fermented coconut (lactobacillus/ Cocos nucifera fruit extract) (HANDAYANI et al. 2008 ), fermented chili (lactobacillus and Capsicum frutescens fruit ferment extract) (Xu et al. 2021 ), fermented pumpkin (lactobacillus/pumpkin fruit ferment filtrate) (Park et al. 2019 ) offer the ability to replace conventional synthetic preserving agents, stimulate blood circulation for strengthening of hair and scalp, and exfoliating properties, respectively. Organic acids viz. citric acid, lactic acid, glycolic acid, etc. produced by microbial fermentation processes are the most versatile ingredients and used in pharmaceutical and cosmetic industries (Vandenberghe et al. 2018 ). Hemisqualane, made via fermentation of sugarcane, is a sustainable and naturally derived alternative to cyclomethicone, a type of liquid silicones used as an emollient, stabilizer, etc. in cosmetics products and associated with bioaccumulation and toxicity (McPhee et al. 2014 ). Fermented ingredients are considered new-generation active ingredients in cosmetic startups (Ecovia Intelligence 2020a ) “Hands on veggies” in Austria is one such start-up working on organic ferments and encouraging pure plant-based products and recyclable packaging made of organic plastic.
Recombinant DNA (rDNA) technology is a process for the production of a wide range of pharmaceutical compounds from genetically manipulated microbe/plant/animal cells. Examples of rDNA technology are lipids/oil from algae, proteins derived from stem cell lines, stabilized enzymes for topical application, etc. (Rinaldi 2008 ). Microalgae is a rich source of fatty acids, lipids, proteins, amino acids, and algal extract from Spirulina that has been used as an antioxidant, and anti-aging agent (Miranda et al. 1998 ; Bermejo et al. 2008 ; Koh et al. 2017 ). The ability of stem cells to regenerate old damaged cells is remarkable and various plant stem cells showed a great effect in the treatment of wrinkles, improved skin elasticity and smoothness, and improved activity collagen production (Bazylak and Gryn 2015 ; Miastkowska and Sikora 2018 ). Different peptides, produced via biotechnological processes, are shown to trigger fibroblast for collagen synthesis, stabilize trace elements needed for wound repair, and inhibit neurotransmitters at the neuromuscular junctions (Weidmann and Craik 2016 ; Bae et al. 2020 ). The use of marine biotechnology in fighting against aging, inflammation, generation of free radicals, and degradation of skin cells is gaining momentum (S. Babitha 2011 ; Wu and Lu 2011 ). A product Homosta-Sea from Atrium Biotechnologies combines ingredients from four different parts of algae as Homeo-Shield, Homeo-Age, Homeoxy, and Homeo-Soothe and is used as a “marine solution for skin homeostasis.” Biotechnology also has an important role in the development of bio-based polymers for replacing plastic with bioplastic for recyclable and biodegradable cosmetic packaging and contributes toward sustainable global growth (Degli Esposti et al. 2021 ).
Bio-based cosmeceuticals
When a pharmacy meets cosmetics, it is known as cosmetic pharmaceuticals or cosmeceuticals. Cosmeceuticals have both cosmetic and therapeutic value, but this term is a marketing term and does not have a legal meaning. Common ingredients present in cosmeceuticals are listed in Table 3 . Some ingredients are permitted in cosmetics at low concentrations while are classified as drugs at higher concentrations. As a consumer, we want to have multiple benefits from a cosmetic product. Imagine you apply lipstick or lip balm, and in addition to great color and smell, it rejuvenates or regenerates your cracked lips. Developing such skin-care formulations along with consumer therapeutics benefits is the need of today’s cosmetic industry. However, cosmeceuticals are low-risk products that have defined skin benefits as per cosmetic indications. They are categorized based on the etiology of the target conditions such as skin lightening, sunscreens, scar reducing, antioxidants, anti-aging, etc. Rice bran, one of the most abundant agricultural by-products, when fermented with mixed cultures of Aspergillus oryzae and Rhizopus oryzae was confirmed as an anti-pigmentation and anti-aging agent for cosmeceutical potential (Abd Razak et al. 2017 ). Jerusalem artichoke extract or powder fermented with P. rhodozyma for astaxanthin synthesis was studied because of its anti-inflammatory, sun proofing, anti-aging, antioxidative, and immune-boosting functions (Jiang et al. 2017 ). Aronia melanocarpa leaf and fruit extract are used in cosmetic products for skin conditioning, skin whitening, anti-inflammatory, and collagen-boosting properties (Cujic et al. 2017 ; Lee and Ryu 2018 ). Novel cosmeceutical delivery systems that act as smart carriers for encapsulation or attachment of therapeutic ingredients need to be explored. Arora et al. 2012 (Arora et al. 2012 ) listed some novel cosmetic delivery systems viz. vesicular delivery system, particulate systems, emulsion delivery systems, and delivery devices such as iontophoresis and cosmetic patches which can help to modulate the skin barrier or administer active ingredients in the skin while minimizing systemic involvement. Some of the commercially available smart delivery systems are microsponge, unispheres, natipide II, Orgasol, Elespher, etc. (Arora et al. 2012 ). Herbal extracts or ingredients used to cure diseases are explored in the literature to usher in a new era of cosmeceuticals (Patil et al. 2017 ).
Microbiomics: targeting skin microbiome with biocosmetic
Our skin microbiota is comprised of millions of microbial species including, bacteria, yeast, fungi, and viruses, and maintaining the right balance between them is required for healthy skin (Byrd et al. 2018 ). Several skin disorders have been associated with alteration in microbial communities, such as atopic dermatitis with Staphylococcus aureus species (Kim et al. 2019 ), acne with Cutibacterium acnes , and Staphylococcus epidermidis (Fournière et al. 2020 ), dandruff with Malassezia species (Saxena et al. 2018 ), psoriasis with an increase in Firmicutes (Koper et al. 2020 ), etc. Some microbes modulate the body odor in particular auxiliary odor by decomposition of apocrine sweat and foot odors from eccrine sweat (James 2020 ). A range of cosmetic products has been developed to improve or alter our skin microbiota. The first cosmetics developed to study the effect of the microbiome on auxiliary odor were deodorants. Since then, new approaches were exploited to treat acne, dermatitis, or even dandruff. Dr. Chris Callewaert created “Dr. Armpit,” a communication platform to discuss research on the microbiology of body odors. The majority of the skin microbiota are still not studied due to difficulty in their isolation. Very specific symbiotic living conditions make them almost impossible to grow in lab conditions. Bacterial species with high impact on dry, oily, and normal skin types are Cutibacterium acnes , Staphylococcus Epidermidis , and Corynebacterium Kroppenstedtii , respectively (DSM 2018 ) . Today, diversity among skin-related bacterial communities is widely explored in forensic studies as well.
The current skincare market analyses four approaches i.e., removing bacteria, feeding good bacteria (prebiotic), adding good bacteria (probiotics), and adding by-products of bacteria (postbiotic). Often there are hurdles while developing a cosmetic formulation on probiotics, i.e., living bacteria due to the maintenance of their integrity and survival, their lifetime, and impact on skin microbiota and hence “probiotic”–containing products are either lysate or fermentation extracts. Elles Magazine, 2015 named probiotics as “bacteria which make us beautiful.” Vogue, 2016 claimed probiotics as an “invisible anti-aging shield.” Elle Magazine, Madame, Figaro, Shape, and Vanity Fair, 2017 claimed probiotics as a “solution for beauty.” Some pre, pro, and postbiotic beauty products occupying the cosmetic market are The One Restorative Cream, Symbiome, Bio Barrier Nourishing Oil, Biophile, Vitamin C Probiotic Polish, Osea, Moisturizer, Mother Dirt, Pre + Probiotic Daily Mist, Marie Veronique, Revitalise and Glow Serum, Aurelia Probiotic Skincare, etc. There are plenty of opportunities to create, redefine or reposition the established products. Major players who turned their attention toward developing microbiome-based cosmetics are Unilever, Johnson and Johnson, Amore Pacific, L’Oreal, Bebe & Bella, Esse, and Mother Dirt. Some of the key challenges associated with a flourishing market are lack of standards and regulations, lack of clinical evidence, and the limit on microorganisms in cosmetic products (Dreher-Lesnick 2018 ; Boxberger et al. 2021 ).
Cosmetic use of organofilization technology
Organoclay is defined as the combination of clay minerals (phyllosilicates) and organic surfactants (quaternary alkylammonium salts and others). Organoclays are formed when the hydrophilic surface is modified by introducing long-chain organic compounds (organofilization) for high sorption of organic pollutants and reduced volatile organic compounds (VOCs) (de Paiva et al. 2008 ). In cosmetic use of organofilization technology, organically modified clays and active agents are used to develop biocomposites/nanocomposites with better quality and shelf life. Salmiņa et al. 2021 (A. Salmiņa, R. Ozola-Davidane 2021 ) proposed anthocyanin-based composites in biocosmetics by intercalating anthocyanin into montmorillonite. Anthocyanins are water-soluble natural pigments with antioxidant activity and play a vital role in many health disorders such as diabetes, cardiovascular diseases, cancer, etc. But these molecules are unstable with changes in pH, temperature, and light and limiting their usage (Georgiev et al. 2014 ; Santos-Buelga and González-Paramás 2018 ). Once combined with clay minerals, these pigments can be stabilized and used for a wide range of applications such as food, biocosmetics, and biomedicine (Ribeiro et al. 2018 ; Rose et al. 2018 ). A recent study investigated the use of polylactide (PLA)-organoclay nanocomposite for cosmetic packaging, a biodegradable solution for conventional petroleum-based plastic (Connolly et al. 2019 ). PLA is produced industrially (NatureWorks ® LLC, USA) through fermentation of sugars (starch, sugarbeet, and corn) however factors such as brittleness, cost, and poor gas barrier properties limit the use of these polymers. Depending on the structure of clay material and its dispersive nature, these composites may confer more strength and enhanced barrier properties due to the compatibility of hydrophilic organoclay with polymeric matrix (Prakalathan et al. 2012 ; Darie et al. 2014 ; Murariu and Dubois 2016 ). The use of nanoclay and organoclay-based composites in cosmetic packaging and migration studies related to the release of these materials into cosmetics is still limited and needs further investigations.
Hydrotalcite (HT) or layered double hydroxide (LDH) is an anionic clay, a versatile substance and “darling of the science world.” Its name is derived from its resemblance with talc and high water content. HT hybrids offer drug encapsulation with high loading capacity, non-toxicity, controlled and targeted delivery, biocompatibility, and desired superficial accumulation of active ingredients through the skin (Ryu et al. 2010 ; Jin et al. 2020 ; Kesavan Pillai et al. 2020 ). It helps to enhance rheological properties as well as stabilization of the cosmetic formulation. Encapsulating the actives in HT extends their release time and, in turn, increases the effectiveness and tolerance. HT is an anion exchange material and its guest–host type structure offers exceptional intercalation chemistry for a wide range of applications (Kesavan Pillai et al. 2020 ). In sunscreens, HT hybrids/LDH are used as carriers for controlled delivery of chemicals (UV absorbers/filters), which on direct contact with skin may cause skin allergies or irritation (Perioli et al. 2006 , 2008 ; Costantino et al. 2008 ; Li et al. 2017 ; Egambaram et al. 2019 ). Due to its versatility, HT hybrids can also be used as a smart carrier delivery system for the transfer of natural pigments, which are essential for skin but suffer from disadvantages such as low stability, skin irritations, pH and temperature limitations, and light irradiations (Bernardos et al. 2019 ).
Identify, predict, and screen bioactives with artificial intelligence (AI)/machine learning (ML)–based approaches
AI/ML-based tools like SkinBug (Jaiswal et al. 2021 ) help predict the reaction between active molecules and skin microbes and determine whether it will be bio-transformed or metabolized to other products to ensure product safety. AI is not only used for the selection of bioactive ingredients, but it also has a bigger role in the selection of formulation process and in offering customized cosmetic formulations. An Irish biotech company “Nutritas” uses genomic technology and AI to identify and extract unique bio-peptides or bioactive peptides for health benefits. Their focus is to identify them first using AI and then extract these ingredients to unlock health potential. Beiersdorf, a company behind Nivea and La Prairie, announced a partnership with a biotech firm “ Insilico Medicine” for discovering new active ingredients for cosmetics using AI. AI-powered skin analysis uses face-mapping technology and looks for signs of aging, pigmentation, skin tone, elasticity, skin texture, moisture, brightness, etc., and allows shoppers to suggest the right cosmetic product for specific skin and health requirements. An MIT-founded AI-driven start-up “Atolla” (Deanna Utroske 2021 ) uses AI to develop skin-specific customized serum and claims its ingredients to be vegan, cruelty-free, without added fragrance, allergy-friendly, and responsibly farmed. Some of the principal, supporting actives and base ingredients in such formulations are coconut extract, ginger root extracts, cactus extract, prickly pear extracts, rumex occidentalis extract, alpha arbutin, betaine, diacetyl boldine, willow bark, apricot kernel, oil, safflower seed oil, etc. All top beauty brands MAC, Estée Lauder, Clinique, L’Oréal, and Neutrogena dedicate their resources to AI to compete in the global market and anticipate emerging trends. Using big data and machine-learning algorithms, beauty apps such as ModiFace, CareOS Technologies’ Smart Mirror, FOREO For You provide customized skincare products as per skin concerns and goals.
Personalized cosmetic with 3D bioprinting
3D bioprinting or additive manufacturing is a process where tissue-like structures are built in record time to mimic the natural tissues of our body. It enables the production of the patient’s cells, which are perfectly compatible during skin grafting or even during organ replacement (Velasquillo et al. 2013 ; Yan et al. 2018 ). Bioinks used in bioprinters are biocompatible materials such as polysaccharides, protein, synthetic polymers, etc., and depending on the application personalized treatment can be developed, e.g., depigmented surface for treating vitiligo can be treated with growth factors, stem cells, and cytokines (Czajkowski 2011 ). Skin scars, often hyperpigmented, can be treated with bioprinting by incorporating a specific amount of melanocytes. Inducing hair follicle regeneration using stem cells has also been demonstrated in the literature (Weinberg et al. 1993 ).
The first living organ to be introduced in the market is a human skin made of all three layers. The key to success i.e., how to mix the individual bio components is patented by CIEMAT (Center for Energy, Environmental and Technological Research) (Cubo et al. 2017 ). Printed skin was successfully used to treat burns and surgical wounds. This technology is revolutionizing the beauty industry as a game-changer by attracting interest from cosmetic giants such as L’Oréal, BASF, and Procter & Gamble from the skincare industry. Unlike the current facemask, where valuable serum leaks everywhere, facial recognition technology like MaskiD helps us to scan and print 3D sheet masks for applying on your face perfectly without any leakage. “Mink,” the first 3D printer for wearable make-up, allows users to choose image color either from the web or in the real world and print that color into blush, eye shadow, lip-gloss, etc. A new development in 3D cosmetics is “human hair” that makes the lives of cancer patients easier. L’Oreal and French biotech company Poietis signed an agreement to bioprint hair follicles capable of growing to attack baldness. Another application is cosmetic packaging such as Collcap Packaging with Objet 30 Pro 3D printing system for cosmetics and perfumes.
Role of industries in offering natural ingredients
Food and marine industry.
Cosmetic ingredients are categorized into active ingredients, excipients, or additives. The marine industry opened up many possibilities for the isolation of active ingredients, and nearly 25,000 new bioactive compounds have been identified (Alves et al. 2020 ). Some of them are high commercial value market products used in pharmaceuticals and cosmetics (Martins et al. 2014a ). Marine bacteria, micro-algae, macro-algae, and halophytes are the major source of compound isolation (Lopes et al. 2016 ). As listed in Table 4 , the bioactive compounds extracted from marine organisms are used for treating skin-related issues. A few marine ingredients are used as excipients and additives in cosmetic formulations for preservatives, essential oil, antioxidants, and dyes (Guillerme et al. 2017 ; Thiyagarasaiyar et al. 2020 ).
Dietary ingredients when used in cosmetics are referred to as food-based cosmetics or healthier cosmetics or nutricosmetics. Detoxifying effects and safety of food ingredients are the major motivations behind this industry. Nutrients present in food such as minerals, vitamins, lipids, proteins, carbohydrates, polyphenols, etc. either provide energy or repair the body for proper functioning. Some dietary ingredients such as vitamins, and carotenoids act as antioxidants, neutralize the free radicals generated during irradiation with UV or during oxidative stress, and prevent skin aging (Akbar et al. 2020 ). Bioactive peptides, derived from food proteins, offer antioxidant, antimicrobial, immunomodulatory, cytomodulatory properties, and other metabolic effects. Once absorbed, they modulate the physiology of systems and improve skin permeability, solubility, stability, and interaction with cell surface receptors. A bioactive peptide can be a signal peptide, carrier peptide, or a neurotransmitter inhibiting peptide. Their development through enzymatic hydrolysis and microbial fermentation has challenges such as stability, susceptibility to further hydrolysis, and reproducibility due to the uncontrollable nature of metabolic activities in microbes respectively. Several companies e.g., PharmaSpecial®, Galena®, Biotec®, Lipotec®, and Silab® are investing their efforts and money developing innovative bioactive peptides. Despite many cosmetic ingredients from marine or food sources, only a few are commercialized because of the need to optimize the process for its production and purification. Furthermore, cost analysis and scalability of the process to ensure the feasibility and performance test to study the effectiveness and safety of cosmetic formulations are needed for large-scale applications.
Textile industry
With the growing trend toward a greener and sustainable market, the textile industry is also looking for innovative ways to create fabric, which can transfer active substances on contact with the skin. The idea is to impart natural ingredients to the fabric so that the wearer’s skin can be moisturized, slimmed, energized, or protected against UV radiations (G and S 2018 ). To achieve this, microencapsulation technology appears to be a suitable method because of its versatile and flexible nature. In this technology, active ingredients are encapsulated in microcapsules, which are tiny particles of liquid/solid material (core) surrounded by a continuous film of polymeric materials (coating), and act as a barrier for the controllable release of core content (Cheng et al. 2008 ; Martins et al. 2014b ; Carvalho et al. 2016 ; Nandy et al. 2020 ). The natural movement of the body, pH, temperature, humidity triggers microcapsules in cosmetotextiles and breaks up to liberate cosmetic ingredients to the skin. Cosmetotextile is associated with toxicity due to polymeric coatings and hence fabric with natural/bio-based microparticles known as dermotextile replace cosmetotextile. Dermotextile offers deep penetration of active ingredients into tissues and protects/preserves skin and hair. Developing odor-resistant textiles, incorporation of fabric with vitamins and minerals are some of the innovative ideas of the future textile industry. The hydrophobic odor molecules entrap in the cavities of cyclodextrins and wash up during laundering (Andreaus et al. 2010 ). Natural materials used for impregnation of textile are plant derivatives such as aloe vera, padina povonica, flowers, fruits, essential oils, animal derivatives such as chitosan, squalene, and sericin, and synthetic materials such as iron oxide, ethanediol, zinc oxide, and zinc nanoparticles (G and S 2018 ). The cosmetic finish is not just for the wearable fabric but also applicable for interior textile products such as curtains, bedsheets, pillow covers, carpets, etc. A neck warmer, debuted in 2015 by L’Oréal and Roxy, claimed for skin nourishing is made of microcapsules that contain anti-inflammatory ingredients such as apricot oil, shea butter, marine-christe extract, and antioxidant vitamin E (Mellage 2016 ). Beiersdorf launched Nivea Q10 plus firming and shaping shorty said to encapsulate Q10 ingredients (Knott et al. 2015 ). The global market for cosmetotextile is expanding with products in the market that prevent the skin from aging, wrinkles, brown age spots, and UV radiations.
Biopolymer industry
The diverse range of synthetic and natural polymeric materials are used in cosmetics for applications such as film former in hair fixatives, rheology modifier in gel, emulsifier and waterproofer in sunscreens lotions and makeup, hair and skin conditioner, emollient in lotions, pigment disperser, emulsion stabilizer, thickener, and as micropore sponge for controlled release of ingredients (Lochhead 2007 , 2017 ). Synthetic polymers are classified into homopolymers viz. polystyrene, polyisobutene, etc., copolymers viz. acrylamide, acrylate, simple vinyl polymers, etc., and cross polymers viz. adipic acid/diethylene glycol/glycerin, etc. Natural polymers can be homopolymers i.e., mono, di, and polysaccharides, heteropolymers composed of different monosaccharides and their derivatives, large groups of complex polysaccharides attached to proteins, protein type polymers, and nucleic acid. Other subcategories are silanes or siloxane-based polymers. Natural polymers are used as thickeners, structuring agents that give rigidity, hair products are starch, gelatin, agar, alginates, polysaccharides, pectin, xanthan, or guar gum, hydrolyzed proteins, cellulose derivatives, natural waxes, long-chain fatty alcohols, and triglycerides (Aggarwal et al. 2020 ). Biopolymers serve as a delivery system for natural antioxidants, antimicrobial and anti-acne ingredients such as hydrogel based on collagen chitosan, gellan gum (Lochhead 2017 ; Das and Giri 2020 ; Mitura et al. 2020 ) micro, nano, and carboxymethylcellulose with crosslinking agent alginate encapsulating bioactive compound from Chlorella vulgaris, etc.(Morais et al. 2020 ).
Rubber industry
Natural rubber latex obtained from Hevea brasiliensis is a milky fluid with polymer cis-1,4-polyisoprene and various plant proteins and is known to possess antibacterial and antiviral properties for plant protection. Natural rubber latex has high strength, tensile strength, easy film-forming, and the capability to stretch without breaking. Compounds from natural rubber are exceptionally flexible, corrosion-resistant, and electric insulators. Natural rubber is positioned as a cleaning sponge in the cosmetic industry. The Thailand Center for Excellence and Life Sciences (TCELS) claimed to transfer innovation and technology to companies for several skincare products with rubber latex extract in collaboration with other leading universities (Andrew McDougall 2014 ). In a study by Oya et al. 2000 (Oya et al. 2000 ), natural rubber latex is used for making a cosmetic Eye Putti® for treating blepharoptosis and showed satisfactory results. In another study by Pichayakorn et al. 2013 , a peel-off mask was prepared from natural rubber latex (Pichayakorn et al. 2013 ). However, several latex-derived products such as face and body paints, eyelash adhesives, hair bonding adhesives, eyeliner, etc., are associated with allergic reactions and need FDA approval before commercialization (Zaza et al. 1994 ; Smith et al. 1998 ; Farnham et al. 2002 ).
Waste industry
Recycling waste is an excellent approach in the new sustainable cosmetic product development to address consumer needs. Different categories of agro-industrial residues e.g., food by-products, vegetable peels, groundnut oil cake, soyabean oil cake, coconut oil cake, ashes, clays, seeds, stalk, leaves, roots, husk, bagasse, molasses, etc., are explored for extraction of natural ingredients. Such wastes are rich in healthy compounds and produced in heavy volumes due to consumption by a large population. Ingredients extracted from food waste such as orange peels, coffee grounds, broccoli leaves/stems have been used for developing cosmetics groundnut scrub, face cream, and peel-off masks (Pacifico et al.). The food processing industry generates an enormous amount of non-edible waste, which has safe and effective ingredients to create biocosmetics. Sugarcane ash, the byproduct of the sugar industry produced after burning sugarcane bagasse, has become a source for a new ingredient “silica,” widely used in the personal care industry. Aprinnova, a leading manufacturer of natural and sustainable emollients and silicone replacements, claims that “Biosilica” can be used as a sustainable alternative to silica used in foundation, creams, lotions, etc. Research studies demonstrated the mesoporous structure of biosilica for high adsorption capacity (Rovani et al. 2018 ), sebum control, and as a photochromic pigment filler (Chindaprasirt and Rattanasak 2020 ). Oil palm leaves and leftover residue contain antimicrobials such as alkaloids, tannins, and flavonoids and are used in producing soap bars (Febriani et al. 2020 ). Sugar beet pulp, waste during extraction of sugar from sugar beet and is usually sent for animal feed, is used to produce 2G-lactic acid via continuous fermentation by Bacillus coagulans used in a variety of applications such as plastic replacement, medical sutures, controlled release of drugs, etc. (Febriani et al. 2020 ). Table 5 lists some of the waste residues widely used for the extraction of bioactive. The Biowaste program funded by European Commissions Department of Agriculture aims to explore agricultural products with high protein and oil-containing residues under a project “Apopros,” and products derived from fruits and vegetables under another project called “Transbio” (Giorgio Dell’Acqua, Ph.D. 2017 ). The challenging part is to extract and purify the ingredients without compromising the stability of ingredients in an environmentally friendly manner with a high yield. In some studies, the toxic nature of these wastes has also been demonstrated, e.g., coffee silverskins, the main waste of the coffee-roasting industry, have undesirable compounds such as phytosterol-oxidized products (POP) and ochratoxin A (OTA). OTA has been classified as a possible carcinogen by the International Agency for Research on Cancer (IARC) and is known to induce nephropathy, renal toxicity, and immunosuppression (Ferraz et al. 2010 ; Kumar et al. 2012 ). Its content is three times higher than permissible limits in coffee silverskin and is a risk to human safety (Toschi et al. 2014 ). Agro-waste can also be contaminated with fungal toxins, pesticides, and industrial chemicals and hence they need careful neutralization before putting it for human use (Abou Fayssal et al. 2020 ).
Beauty and personal care: post-COVID-19 outbreak
The coronavirus pandemic has affected every facet of the economy and redirected our minds to create a better and healthier world. During the pandemic, the cosmetics industry was severely affected due to the disrupted supply of raw materials, production processes, marketing, and distribution of finished products. The impact of COVID-19 on the cosmetic industry was discussed in European and North American editions of the Sustainable Cosmetic Summit 2020 and emphasized the need for a circular economy in the beauty and personal care industry (Deanna Utroske 2020 ). During this summit, industry experts and executives discussed several approaches, such as a change in existing cosmetic formulations, ingredients, growing consumer sentiments toward natural products, and sustainable packaging. The key issues of the summit were (1) cosmetic products are directed to interact with human virome to maintain good skin health, (2) the growing consumer trend is toward natural ingredients to boost skin immunity and hence the beauty industry must leverage the products with anti-inflammatory and skin immune-boosting ingredients, (3) changing sustainability agenda in the cosmetic industry, implementing good sustainability practices in the cosmetic industry, and choosing the product sustainable “inside and out,” (4) disruption of supply for raw material due to higher freight costs, longer transportation times, and quarantine measures at ports during the pandemic is encouraging the operators to go regional for natural ingredients, (5) upcycling food ingredients e.g., a French firm developed a product for eye puffiness and dark circles from discarded avocadoes, Givaudan Active Beauty launched Koffee’ Up™, an alternative to argan oil, from spent coffee grounds, Rahn AG and Cargill beauty is upcycling pumpkin seeds and leftover lemon peel for skin care products (Ecovia Intelligence 2020b ; Deanna Utroske 2020 ), (6) sustainable palm oil and rainforest ingredients, which are environmentally and socially acceptable, (7) the social value of cosmetics by promoting social inclusivity and multiculturalism, (8) retailing implications due to the ongoing shift to digital, and (9) marketing to the post-COVID consumer by focusing on health-related product development.
Factors fueling the growth of the cosmetic industry before COVID-19 were modern lifestyle coupled with aesthetic appeal plus highlighting/promoting the brand on various media platforms. However, with the coronavirus outbreak, the cosmetic market segment experienced a severe downfall due to lockdown and the worldwide closing of offline stores (Wood 2020 ). Several factories shifted to making hand sanitizers and cleaning agents to ensure their survival (Miller 2020 ). Although the COVID-19 outbreak forced the world to close borders, it kickstarted the idea to build back better with increasing demand for natural and healthier products. As per a recent survey, consumers perceive healthy skin as one of the resistant factors against environmental stress, and hence natural and organic ingredients are gaining more popularity after the pandemic (Intelligence. 2020 ). Suppliers of aloe vera, grapeseed oil, tea tree oil, CBD oil, palm oil reported a spike in demand for natural ingredients (Imogen Matthews 2019 ; Intelligence. 2020 ), and research studies in the past also demonstrated the importance of natural ingredients in personal care products and showing how nature can heal, smoothen, restore and support us to cope with life’s demands. For example, aloe vera, termed as the plant of immortality by ancient Egyptians, has both antibacterial and antiviral properties and is used in a variety of cosmetic products (Long 2016 ). Eucalyptus oil has virus-fighting properties, and essential oil-based ingredients such as balm, inhalers, and diffusers reduce virus transmission (Sienkiewicz et al. 2011 ). Grapeseed oil strengthens connective tissues along with its anti-inflammatory, antioxidant, and healing properties (Sumaiyah and Leisyah 2019 ). Green tea and black tea molecules were found to inhibit virus entry (Carneiro et al. 2016 ; Mahmood et al. 2016 ; Ohgitani et al. 2021 ). Palm oil, palm kernel oil, and its derivatives, present in almost 50% of the cosmetic products, are a rich source of vitamin E, carotenoids, squalene, lauric acid, etc., and are used as antibacterial liquid soap (Nainggolan and Sinaga 2021 ). Tea tree oil is a popular natural ingredient used in shampoos, skin and nail creams, massage oils, and laundry detergents. It is a known antiseptic agent used against bacteria, fungi, viruses, and mites (Larson and Jacob 2012 ). Tea extract and lemongrass, rich with phenolic compounds and antioxidants, are used for producing hand sanitizers with enhanced antimicrobial efficacy (Rana 2021 ). Post-Covid, Australian companies observed high demand for lemon oil, and 180,000 new lemon myrtle ( Backhousia citriodora ) and anise myrtle ( Syzygium anisatum ) trees were grown to ramp up the production (Intelligence. 2020 ). Consumer sentiments toward hygiene, wellness, and safety are going to cause a longer shift in their spending priorities and trade down to value-for-money products. From the current scenario, it is predictable that sanitation and immunity-booster products will continue to occupy the high demand with a focus on family and self-care.
Future outlook
Zero waste cosmetic.
Adopting a “zero waste” policy is an important step to stop cosmetic waste from entering the human body through a polluted environment. Implementing zero waste and zero CO 2 emission policies in the sourcing, extraction, production, and packaging processes of cosmetics will push the idea of “Green Growth.” Recycling industrial waste should not be an option rather an obligation to achieve a circular economy. Several make-up beauty brands such as Alima Pure, Axiology, The lip bar, Aether beauty, RMS beauty are adopting this policy and prioritizing cruelty-free, vegan, non-GMO ingredients and recyclable glass or fiber, paper-based packaging. The environmental impact of conventional cosmetics made of microbeads, microplastic, and triclosan is huge and calls for serious attention (Anagnosti et al. 2021 ). These smaller size particles are impossible to clear out of the ocean, become a part of the natural cycle, and enter the food chain. VOCs in fragrances and sprays cause smog and air pollution (Steinemann et al. 2021 ). The UV filters avobenzone, octocrylene, and oxybenzone in sunscreens are known to enter systemic circulation, contaminate the environment and crop productivity (Matta et al. 2019 ) (Zhong et al. 2020 ). Pesticides and chemicals sprayed on crops, which are used for the extraction of ingredients pose severe damage to human health (Kuang et al. 2020 ). Ultrafine crystalline silica (SiO2) nanoparticles used in cosmetics alter genes and cause severe mutations. There is strong evidence that nanoparticles reach our brain through inhalation and cross the blood–brain barrier (Oberdörster et al. 2002 ). Wet wipes, used for cleaning our face, removing make-up, and cleaning baby bum are flushable and eventually reach oceans. Sea creatures such as turtles die when they consume these wipes (Ó Briain et al. 2020 ). BHA and BHT, synthetic antioxidants used as cosmetic preservatives, enter aquatic species and cause genetic alteration (Lundebyea et al. 2010 ). Although the above-mentioned environmental offenders raise serious concerns and cosmetic brands are committed to recycling cosmetic waste, progress is still very slow because recycling is not an easy one-step solution. Recycling waste is more complicated than it may seem and is associated with several challenges (1) manual sorting and segregation of hazardous and non-hazardous waste where technology does not replace the decision-making ability of humans, (2) exposure of occupational workers to dust and airborne contaminants, (3) safety of workers toward potentially toxic substances and educating them about recycling equipment, and (4) cosmetic packaging is a mixture of different materials, making hard to recycle. A long-term sustainable solution is to switch from conventional to waste-free natural cosmetics.
Transformation to beauty and wellness with new generation natural ingredients
Moving away from illness, treatment, quick fix, reactivity, and obligation of current cosmetics toward the vitality, prevention, long-term effectiveness, proactivity, and experience offered by biocosmetics is the current consumer mindset. The cosmetics sector offers tremendous future opportunities for startups involved in the formulation and customization of products as per individual skin characteristics, replacing fossil-based ingredients with natural, clean, vegan, sustainable, and high efficacy ingredients, encouraging social inclusivity, and niche-focusing products based on gender, age group, price, etc. The technological advancements, as discussed, offer ways to produce natural, and effective ingredients with controlled and targeted delivery formulations. Industries, which offer bio-based raw materials such as agricultural, food, marine, waste industry, biopolymers, bioplastics, natural rubber, are helping to reduce carbon footprints and creating a recyclable and biodegradable regime for cosmetic products. Today’s ingredient-savvy consumer is demanding a combo of beauty and wellness and prioritizing quality over quantity. A new concept “beauty inside out” requires proactively looking into new-generation natural ingredients such as green surfactants and green materials with the aid of technological advancement. For example, natural deep eutectic solvents (NADES), the “natural” solution, is a new and exciting technology for the production of eutectic natural solvents where metabolites are solubilized inside the plant (Jeliński et al. 2019 ; Osowska and Ruzik 2019 ). Protective and defensive compounds extracted from marine algae (Stiger-Pouvreau and Guerard 2018 ), anti-aging, antioxidant, anti-wrinkle, skin-firming ingredients using plant-cell culture (Georgiev et al. 2018 ), novel cosmetic delivery systems (Patravale and Mandawgade 2008 ), stem cell-derived cosmetic products (Zarei and Abbaszadeh 2018 ), and novel anti-infective short antimicrobial peptides, as cosmetic ingredients (Rahnamaeian and Vilcinskas 2015 ) are some of the recent research addressing this area. Many natural ingredients with cosmetic potential are difficult to penetrate the skin barriers, showing poor bioavailability, low solubility, and uncontrolled release (Dini and Laneri 2021 ); therefore, novel methods of encapsulating these natural bioactive substances with immediate and tangible results need further exploration. Cosmetic use of organoclay is also limited, with good color retention for nail paints, eye shadows, and lipstick, and offers a tremendous future opportunity to explore other applications.
Commitment to sustainability
With the rise in demand for natural and greener products, concerns arise toward sustainability. The production of grains (oat, barley, and wheat) based extracts used in face creams and foundations have concerns with water pollution (Almendinger et al. 2020 ; Hwang et al. 2020 ). Palm kernel oil-based products such as lipstick, soap, shampoo, etc., have concerns about massive deforestation and plant and animal diversity, and hence cosmetic companies are keen to search for an alternative such as coconut oil and babassu oil (Kaupper et al. 2020; Whiffin et al. 2016; Martinez et al. 2017). Sustainability is multidimensional and must address all economic, environmental, and social aspects. Company sustainability should have a “think green” holistic approach i.e., right from sourcing the raw material to the extraction of bioactive ingredients, formulation processes and finish, product testing, final packaging, and ultimately the marketing strategies. The “eco-conception approach” reviews all processing steps in terms of resource management, energy utilization, global impact, waste management, waste consumption, quality control, and lowering carbon footprints. Today, many cosmetic products do not deliver what they claim, which is a big disappointment for the customer. To address this problem, transparency on the sourcing of raw material, processing the ingredients as per regulatory guidelines on the use/concentration of ingredients in cosmetic formulations must be achieved that will ensure quality, integrity, and safety of the product. Eco-compatible cosmetic packaging is receiving much attention due to its reuse, recycle, and biodegradable nature; however, it poses a challenge due to packaging often contaminated with cosmetic residues difficult to remove by washing. Compostable packaging replaces plastic packaging with bio-based polymers and bioplastic, but the degradation is affected by the presence of detergents or preservatives detrimental to microbial activity (Asgher et al. 2020 ). Therefore, a correct product design and packaging material need careful consideration based on the intrinsic instability of cosmetic formulation so that the property of a cosmetic product does not change on storage, transportation, or exposure to UV radiations.
Facing challenges of “going green”
Biocosmetics are environmentally friendly, protect our long-term health, nourish and heal the skin naturally, and do not mess up with natural hormone production and fertility. However, gray areas do exist in the biocosmetic industry that needs future exploration: (1) the slowness of natural ingredients obtained from plants and animals, (2) the high cost of biocosmetic products, especially when pure/concentrated bioactive ingredients are to be used in the formulations, make them unaffordable to the majority of the customers, (3) low shelf life and non-vibrant colors with a limited number of shades available, (4) fear of blemishing brand reputation due to risk in the supply chain of raw material across continents, (5) environmental impact of using agricultural-based raw materials, (6) seasonal fluctuation in the desired compound production from plants, and (7) lack of unified international standard guidelines for “green cosmetic producers” and risk assessment approaches. The adverse effect has also been reported in the past from plant extracts (Franca and Ueno 2020 ) and some of the natural substances are found to be non-degradable; therefore, safety analysis and compliance with the green chemistry principle is essential even for biocosmetic products.
Conclusions
The review describes concerns with current cosmetics used in day-to-day life and justifies the move toward green cosmetics. The ingredients used in personal care products are silent killers and responsible for deteriorating human health without their awareness. Cosmetics companies are self-regulated for more than a century and enjoyed enough luxury of self-policing and weak regulations. But now, safety concerns must be addressed, and regulations the same as pharmaceutical drugs must be implemented. Today’s “well-informed” consumer is concerned about the origin, safety, environment, and sustainability. As discussed, the role of advanced technologies and industries in developing natural, sustainable, and healthier cosmetics is crucial to fulfilling consumer demand. Naturality with sustainability is the key to long-term survival for cosmetic companies, and they must set their products accordingly to compete in the fast-paced market. These companies must be accountable for their product designs, safety and efficacy of ingredients, and safe and recyclable packaging.
Availability of data and material
Not applicable.
Code availability
Author (2010) IARC monographs on the evaluation of carcinogenic risks to humans. IARC Monogr Eval Carcinog Risks to Humans 93:9–38. https://doi.org/10.1136/jcp.48.7.691-a
Article Google Scholar
A. Salmiņa R, R. Ozola-Davidane MK (2021) Composites of clay minerals and natural pigments: synthesis, characterization and potential in biocosmetics. In: Dzene Līva, Vircava Ilze (eds) Book of Abstracts: Nordic Clay Meetings. RIGA, LATVIA, p 45
Google Scholar
Abd Razak DL, Abd Rashid NY, Jamaluddin A et al (2017) Cosmeceutical potentials and bioactive compounds of rice bran fermented with single and mix culture of Aspergillus oryzae and Rhizopus oryzae. J Saudi Soc Agric Sci 16:127–134. https://doi.org/10.1016/j.jssas.2015.04.001
Abou Fayssal S, Alsanad MA, El Sebaaly Z et al (2020) Valorization of olive pruning residues through bioconversion into edible mushroom Pleurotus ostreatus (Jacq. Ex Fr.) P. Kumm. (1871) of Improved Nutritional Value. Scientifica (cairo) 2020:1–13. https://doi.org/10.1155/2020/3950357
Article CAS Google Scholar
Aggarwal J, Sharma S, Kamyab H, Kumar A (2020) The realm of biopolymers and their usage: an overview. J Environ Treat Tech 8:1005–1016
Akbar I, Jaswir I, Jamal P (2020) Optimisation of the production of fish gelatine nanoparticles as a carrier for sunflower-derived biopeptide. Int Food Res J 27:171–181
CAS Google Scholar
Al-Saleh I, Al-Enazi S, Shinwari N (2009) Assessment of lead in cosmetic products. Regul Toxicol Pharmacol 54:105–113. https://doi.org/10.1016/j.yrtph.2009.02.005
Riccolo Alexa (2021) The lack of regulation in preventing greenwashing of cosmetics in the U.S. J Legis 47:133
Almendinger M, Rohn S, Pleissner D (2020) Malt and beer-related by-products as potential antioxidant skin-lightening agents for cosmetics. Sustain Chem Pharm 17:100282. https://doi.org/10.1016/j.scp.2020.100282
Alves A, Sousa E, Sousa E et al (2020) Marine-derived compounds with potential use as cosmeceuticals and nutricosmetics. Molecules 25:2536. https://doi.org/10.3390/molecules25112536
Anagnosti L, Varvaresou A, Pavlou P et al (2021) Worldwide actions against plastic pollution from microbeads and microplastics in cosmetics focusing on European policies. Has the issue been handled effectively? Mar Pollut Bull 162:111883. https://doi.org/10.1016/j.marpolbul.2020.111883
Andreaus J, Dalmolin MC, De Oliveira IB, Barcellos IO (2010) Aplicação de ciclodextrinas em processos têxteis. Quim Nova 33:929–937. https://doi.org/10.1590/S0100-40422010000400031
Andrew MCDOUGALL (2014) Scientists develop skin care products from rubber latex extract. In: CosmeticDesign-Asia. https://www.cosmeticsdesign-asia.com/Article/2014/10/02/Scientists-develop-skin-care-products-from-rubber-latex-extract?utm_source=copyright&utm_medium=OnSite&utm_campaign=copyright
Arora N, Agarwal S, Murthy RSR (2012) Review article latest technology advances in cosmaceuticals. Int J Pharm Sci Drug Res 4:168–182
Arroyo GV, Madrid AT, Gavilanes AF et al (2020) Green synthesis of silver nanoparticles for application in cosmetics. J Environ Sci Heal - Part A Toxic/hazardous Subst Environ Eng 55:1304–1320. https://doi.org/10.1080/10934529.2020.1790953
Asgher M, Qamar SA, Bilal M, Iqbal HMN (2020) Bio-based active food packaging materials: sustainable alternative to conventional petrochemical-based packaging materials. Food Res Int 137:109625. https://doi.org/10.1016/j.foodres.2020.109625
Bae J, Kim N, Shin Y et al (2020) Activity of catechins and their applications. Biomed Dermatology 4:1–10. https://doi.org/10.1186/s41702-020-0057-8
Bazylak G, Gryn A (2015) Antioxidant activity and total flavonoid content in variable phyto-stem cells extracts obtained by high-pressure homogenization method and assigned for use in biocosmetics. Planta Med 81:1550. https://doi.org/10.1055/s-0035-1565835
Benson HAE, Roberts MS, Leite-Silva VR, Walters K (2019) Cosmetic formulation: principles and practice. CRC Press
Bermejo P, Piñero E, Villar ÁM (2008) Iron-chelating ability and antioxidant properties of phycocyanin isolated from a protean extract of Spirulina platensis. Food Chem 110:436–445. https://doi.org/10.1016/j.foodchem.2008.02.021
Bernardos A, Bozik M, Alvarez S et al (2019) The efficacy of essential oil components loaded into montmorillonite against Aspergillus niger and Staphylococcus aureus. Flavour Fragr J 34:151–162. https://doi.org/10.1002/ffj.3488
Boxberger M, Cenizo V, Cassir N, La Scola B (2021) Challenges in exploring and manipulating the human skin microbiome. Microbiome 9:1–14
Bråred Christensson J, Karlberg AT, Andersen KE et al (2016) Oxidized limonene and oxidized linalool - concomitant contact allergy to common fragrance terpenes. Contact Dermatitis 74:273–280. https://doi.org/10.1111/cod.12545
Brown VJ (2013) Metals in lip products: a cause for concern? Environ Health Perspect 121:a196. https://doi.org/10.1289/ehp.121-a196
Brunet L, Lyon DY, Hotze EM et al (2009) Comparative photoactivity and antibacterial properties of C60 fullerenes and titanium dioxide nanoparticles. Environ Sci Technol 43:4355–4360. https://doi.org/10.1021/es803093t
Byrd AL, Belkaid Y, Segre JA (2018) The human skin microbiome. Nat Rev Microbiol 16:143–155
Carneiro BM, Batista MN, Braga ACS et al (2016) The green tea molecule EGCG inhibits Zika virus entry. Virology 496:215–218. https://doi.org/10.1016/j.virol.2016.06.012
Carroll IM, Andrus JM, Bruno-Bárcena JM et al (2007) Anti-inflammatory properties of Lactobacillus gasseri expressing manganese superoxide dismutase using the interleukin 10-deficient mouse model of colitis. Am J Physiol - Gastrointest Liver Physiol 293:G729–G738. https://doi.org/10.1152/ajpgi.00132.2007
Carvalho IT, Estevinho BN, Santos L (2016) Application of microencapsulated essential oils in cosmetic and personal healthcare products - a review. Int J Cosmet Sci 38:109–119
Centre for the Promotion of Imports from developing countries (2020) What is the demand for natural ingredients for cosmetics on the European market? | CBI - Centre for the Promotion of Imports from developing countries. In: CBI Lab. https://www.cbi.eu/market-information/natural-ingredients-cosmetics/what-demand
Cervellon M-C, Carey L (2012) Consumers’ perceptions of “green”: why and how consumers use eco-fashion and green beauty products. Crit Stud Fash Beauty 2:117–138. https://doi.org/10.1386/csfb.2.1-2.117_1
Chaudhri N, Soni GC, Prajapati SK (2015) Nanotechnology: an advance tool for nano-cosmetics preparation. Int J Pharma Res Rev 4:28–40
Cheng F, Luozhong S, Guo Z et al (2017) Enhanced biosynthesis of hyaluronic acid using engineered Corynebacterium glutamicum via metabolic pathway regulation. Biotechnol J 12:191. https://doi.org/10.1002/biot.201700191
Cheng SY, Yuen CWM, Kan CW, Cheuk KKL (2008) Development of cosmetic textiles using microencapsulation technology. Res J Text Appar 12:41–51. https://doi.org/10.1108/rjta-12-04-2008-b005
Chiari-Andréo BG, De Almeida-Cincotto MGJ, Oshiro JA, et al (2019) Nanoparticles for cosmetic use and its application. In: Nanoparticles in Pharmacotherapy. pp 113–146
Chindaprasirt P, Rattanasak U (2020) Eco-production of silica from sugarcane bagasse ash for use as a photochromic pigment filler. Sci Rep 10:1–8. https://doi.org/10.1038/s41598-020-66885-y
Connolly M, Zhang Y, Brown DM et al (2019) Novel polylactic acid (PLA)-organoclay nanocomposite bio-packaging for the cosmetic industry; migration studies and in vitro assessment of the dermal toxicity of migration extracts. Polym Degrad Stab 168:108938. https://doi.org/10.1016/j.polymdegradstab.2019.108938
Costantino U, Ambrogi V, Nocchetti M, Perioli L (2008) Hydrotalcite-like compounds: versatile layered hosts of molecular anions with biological activity. Microporous Mesoporous Mater 107:149–160. https://doi.org/10.1016/j.micromeso.2007.02.005
Cubo N, Garcia M, Del Cañizo JF et al (2017) 3D bioprinting of functional human skin: production and in vivo analysis. Biofabrication 9:015006. https://doi.org/10.1088/1758-5090/9/1/015006
Cujic N, Zugic A, Zivkovic J et al (2017) Preliminary safety estimate of cosmetic anti-age creams with chokeberry extract, using in vivo bioengineering techniques. Lek Sirovine 37:41–45. https://doi.org/10.5937/leksir1737041c
Czajkowski R (2011) BRAF, HRAS, KRAS, NRAS and CDKN2A genes analysis in cultured melanocytes used for vitiligo treatment. Int J Dermatol 50:180–183. https://doi.org/10.1111/j.1365-4632.2010.04675.x
Darbre PD, Harvey PW (2008) Paraben esters: review of recent studies of endocrine toxicity, absorption, esterase and human exposure, and discussion of potential human health risks. J Appl Toxicol 28:561–578
Darie RN, Pâslaru E, Sdrobis A et al (2014) Effect of nanoclay hydrophilicity on the poly(lactic acid)/clay nanocomposites properties. Ind Eng Chem Res 53:7877–7890. https://doi.org/10.1021/ie500577m
Darling S (1959) The chemistry of cosmetics. Wis Acad Rev 6:1
Das M, Giri TK (2020) Hydrogels based on gellan gum in cell delivery and drug delivery. J Drug Deliv Sci Technol 56:101586
de Paiva LB, Morales AR, Valenzuela Díaz FR (2008) Organoclays: properties, preparation and applications. Appl Clay Sci 42:8–24
Deanna Utroske (2021) AI-driven skin care startup Atolla expands ingredient portfolio. https://www.cosmeticsdesign.com/Article/2020/11/12/AI-skincare-startup-Atolla-expands-ingredient-portfolio?utm_source=copyright&utm_medium=OnSite&utm_campaign=copyright
Deanna Utroske (2020) 2020 Sustainable cosmetics summit zeros in on circularity. CosmeticOBS
Degli Esposti M, Morselli D, Fava F et al (2021) The role of biotechnology in the transition from plastics to bioplastics: an opportunity to reconnect global growth with sustainability. FEBS Open Bio 11:967–983
Dhillon GS, Kaur S, Pulicharla R et al (2015) Triclosan: current status, occurrence, environmental risks and bioaccumulation potential. Int J Environ Res Public Health 12:5657–5684
Dini I, Laneri S (2021) The new challenge of green cosmetics: natural food ingredients for cosmetic formulations. Molecules 26
Dinwiddie MT, Terry PD, Chen J (2014) Recent evidence regarding triclosan and cancer risk. Int J Environ Res Public Health 11:2209–2217. https://doi.org/10.3390/ijerph110202209
Donnez D, Jeandet P, Clément C, Courot E (2009) Bioproduction of resveratrol and stilbene derivatives by plant cells and microorganisms. Trends Biotechnol 27:706–713
Dreher-Lesnick S (2018) Science and regulation of live microbiome-based products used to prevent, treat, or cure diseases in humans - 09/17/2018 FDA. FDA-NIH Public Work Sept 17(2018):9–12
DSM (2018) Skin microbiome research for a better understanding of specific skin conditions. https://www.dsm.com/personal-care/en_US/about.html
Dwivedi DR (2020) Reducing dependency on petroleum-derived ingredients with plant-based butylene glycols. Azo Mater.
Ecovia Intelligence (2020a) A new generation of natural cosmetic ingredients emerges. In: Cbi. https://biovanitybox.com/blogs/blog/a-new-generation-of-natural-cosmetic-ingredients
Ecovia Intelligence (2020b) Upcycled’ ingredients in cosmetics. Happi
Egambaram OP, Pillai SK, Lategan M, Ray SS (2019) Nanostructured Zn-Ti layered double hydroxides with reduced photocatalytic activity for sunscreen application. J Nanoparticle Res 21:1–12. https://doi.org/10.1007/s11051-019-4488-3
Ekpa Effiong D, Uwah TO, Udofa Jumbo E, Akpabio AE (2020) Nanotechnology in cosmetics: basics, current trends and safety concerns-a review. Adv Nanoparticles 9:1–22
Farnham JJ, Tomazic-Jezic VJ, Stratmeyer ME (2002) Regulatory initiatives for natural latex allergy: US perspectives. Methods 27:87–92. https://doi.org/10.1016/S1046-2023(02)00056-7
Febriani A, Syafriana V, Afriyando H, Djuhariah YS (2020) The utilization of oil palm leaves (Elaeis guineensis Jacq.) waste as an antibacterial solid bar soap. In: IOP Conference Series: Earth and Environmental Science
Ferraz MBM, Farah A, Iamanaka BT et al (2010) Kinetics of ochratoxin a destruction during coffee roasting. Food Control 21:872–877. https://doi.org/10.1016/j.foodcont.2009.12.001
Ferrer-Tasies L, Santana H, Cabrera-Puig I et al (2021) Recombinant human epidermal growth factor/quatsome nanoconjugates: a robust topical delivery system for complex wound healing. Adv Ther 4:2000260. https://doi.org/10.1002/adtp.202000260
Filaire E (2019) New generation skin care active ingredients adapted to modern lifestyles. https://www.premiumbeautynews.com/en/new-generation-skin-care-active,15552#
Fournière M, Latire T, Souak D et al (2020) Staphylococcus epidermidis and cutibacterium acnes: two major sentinels of skin microbiota and the influence of cosmetics. Microorganisms 8:1–31
Franca CCV, Ueno HM (2020) Green cosmetics: perspectives and challenges in the context of green chemistry. Desenvolv e Meio Ambient 53:133–150. https://doi.org/10.5380/dma.v53i0.62322
Fulmer EI, Christensen LM, Kendall AR (1933) Production of 2,3-butylene glycol by fermentation: effect of sucrose concentration. Ind Eng Chem 25:798–800. https://doi.org/10.1021/ie50283a019
Gasdf N, Sasdf K (2018) Integration of cosmetics with textiles: an emerging area of functional textiles - a review. J Text Eng Fash Technol 4:316–318. https://doi.org/10.15406/jteft.2018.04.00158
Georgiev V, Ananga A, Tsolova V (2014) Recent advances and uses of grape flavonoids as nutraceuticals. Nutrients 6:391–415. https://doi.org/10.3390/nu6010391
Georgiev V, Slavov A, Vasileva I, Pavlov A (2018) Plant cell culture as emerging technology for production of active cosmetic ingredients. Eng Life Sci 18:779–798
Giorgio Dell’Acqua, Ph.D. D (2017) Garbage to Glamour: recycling food by-products for skin care. In: Febr. 6, 2017. https://www.cosmeticsandtoiletries.com/research/methodsprocesses/Garbage-to-Glamour-Recycling-Food-by-products-for-Skin-Care-412910643.html
Gomes C, Silva AC, Marques AC et al (2020) Biotechnology applied to cosmetics and aesthetic medicines. Cosmetics 7:33
Guillerme JB, Couteau C, Coiffard L (2017) Applications for marine resources in cosmetics. Cosmetics 4:35
Handayani R, Sulistyo J, Rahayu RD (2008) Extraction of coconut oil (Cocos nucifera L.) through fermentation system. Biodiversitas J Biol Divers 10:100309. https://doi.org/10.13057/biodiv/d100309
Harishchandra BD, Pappuswamy M, PU A et al (2020) Copper nanoparticles: a review on synthesis, characterization and applications. Asian Pacific J Cancer Biol 5:201–210. https://doi.org/10.31557/apjcb.2020.5.4.201-210
Hwang JU, Yang YH, Choe TB (2020) Physiological characteristics of germinated oat (Avena sativa) extract as raw material for cosmetics. Asian J Beauty Cosmetol 18:137–148. https://doi.org/10.20402/ajbc.2020.0012
Hyun JK, Byung ML (2004) Estimated exposure to phthalates in cosmetics and risk assessment. In: Journal of Toxicology and Environmental Health - Part A. pp 1901–1914
Imogen Matthews (2019) There’s high interest in CBD for beauty & personal care. Happi
Intelligence. E (2020) Natural cosmetic ingredients to gain popularity in post-COVID-19 era. Prem. beauty news
Jaiswal SK, Agarwal SM, Thodum P, Sharma VK (2021) Skinbug: an artificial intelligence approach to predict human skin microbiome-mediated metabolism of biotics and xenobiotics. Science 24:101925. https://doi.org/10.1016/j.isci.2020.101925
James AG (2020) The axillary microbiome and its relationship with underarm odor. Skin Microbiome Handbook. pp 79–129
Jeliński T, Przybyłek M, Cysewski P (2019) Natural deep eutectic solvents as agents for improving solubility, stability and delivery of curcumin. Pharm Res 36:116. https://doi.org/10.1007/s11095-019-2643-2
Jiang GL, Zhou LY, Wang YT, Zhu MJ (2017) Astaxanthin from Jerusalem artichoke: production by fed-batch fermentation using Phaffia rhodozyma and application in cosmetics. Process Biochem 63:16–25. https://doi.org/10.1016/j.procbio.2017.08.013
Jin W, Lee D, Jeon Y, Park DH (2020) Biocompatible hydrotalcite nanohybrids for medical functions. Minerals 10:172
Jones O, Selinger B (2017) The chemistry of cosmetics - curious. Aust Acad Sci
Kaličanin B, Velimirović D (2016) A study of the possible harmful effects of cosmetic beauty products on human health. Biol Trace Elem Res 170:476–484. https://doi.org/10.1007/s12011-015-0477-2
Keijok WJ, Pereira RHA, Alvarez LAC et al (2019) Controlled biosynthesis of gold nanoparticles with Coffea arabica using factorial design. Sci Rep 9:1–10. https://doi.org/10.1038/s41598-019-52496-9
Kesavan Pillai S, Kleyi P, de Beer M, Mudaly P (2020) Layered double hydroxides: an advanced encapsulation and delivery system for cosmetic ingredients-an overview. Appl Clay Sci 199:105868
Kim J, Kim BE, Ahn K, Leung DYM (2019) Interactions between atopic dermatitis and staphylococcus aureus infection: clinical implications. Allergy, Asthma Immunol Res 11:593–603
Knott A, Achterberg V, Smuda C et al (2015) Topical treatment with coenzyme Q10-containing formulas improves skin’s Q10 level and provides antioxidative effects. BioFactors 41:383–390. https://doi.org/10.1002/biof.1239
Koh EJ, Kim KJ, Song JH et al (2017) Spirulina maxima extract ameliorates learning and memory impairments via inhibiting GSK-3β phosphorylation induced by intracerebroventricular injection of amyloid-β 1–42 in mice. Int J Mol Sci 18:2401. https://doi.org/10.3390/ijms18112401
Kopelovich L, Perez AL, Jacobs N et al (2015) Screening-level human health risk assessment of toluene and dibutyl phthalate in nail lacquers. Food Chem Toxicol 81:46–53. https://doi.org/10.1016/j.fct.2015.04.011
Koper M, Wozniacka A, Robak E (2020) The intestinal microbiota in psoriasis. Postepy Hig Med Dosw 74:236–246
Kuang L, Hou Y, Huang F et al (2020) Pesticide residues in breast milk and the associated risk assessment: a review focused on China. Sci Total Environ 727:138412
Kulka M (2009) The potential of natural products as effective treatments for allergic inflammation: implications for allergic rhinitis. Curr Top Med Chem 9:1611–1624. https://doi.org/10.2174/156802609789941898
Kumar R, Ansari KM, Chaudhari BP et al (2012) Topical application of ochratoxin a causes DNA damage and tumor initiation in mouse skin. PLoS ONE 7:47280. https://doi.org/10.1371/journal.pone.0047280
Lajis AFB, Basri M, Mohamad R et al (2013) Enzymatic synthesis of kojic acid esters and their potential industrial applications. Chem Pap 67:573–585
Larson D, Jacob SE (2012) Tea tree oil. Dermatitis 23:48–49. https://doi.org/10.1097/DER.0b013e31823e202d
Lee JD, Lee JY, Kwack SJ et al (2019) Risk assessment of triclosan, a cosmetic preservative. Toxicol Res 35:137–154
Lee JM, Ryu MJ (2018) Efficacy of cosmetic materials using aronia melanocarpa leaf extracts. Asian J Beauty Cosmetol 16:179–190. https://doi.org/10.20402/ajbc.2017.0166
Li Y, Tang L, Ma X et al (2017) Synthesis and characterization of Zn-Ti layered double hydroxide intercalated with cinnamic acid for cosmetic application. J Phys Chem Solids 107:62–67. https://doi.org/10.1016/j.jpcs.2017.02.018
Lochhead RY (2017) The use of polymers in cosmetic products. In: Cosmetic Science and Technology: Theoretical Principles and Applications. pp 171–221
Lochhead RY (2007) The role of polymers in cosmetics: recent trends. In: ACS Symposium Series. pp 3–56
Long V (2016) Aloe vera in dermatology-the plant of immortality. JAMA Dermatol 152:1364
Lopes A, Rodrigues MJ, Pereira C et al (2016) Natural products from extreme marine environments: searching for potential industrial uses within extremophile plants. Ind Crops Prod 94:299–307. https://doi.org/10.1016/j.indcrop.2016.08.040
Lundebyea AK, Hovea H, Mågea A et al (2010) Levels of synthetic antioxidants (ethoxyquin, butylated hydroxytoluene and butylated hydroxyanisole) in fish feed and commercially farmed fish. Food Addit Contam - Part A Chem Anal Control Expo Risk Assess 27:1652–1657. https://doi.org/10.1080/19440049.2010.508195
Mahmood MS, Mártinez JL, Aslam A et al (2016) Antiviral effects of green tea (Camellia sinensis) against pathogenic viruses in human and animals (a mini-review). African J Tradit Complement Altern Med 13:176–184
Manke A, Wang L, Rojanasakul Y (2013) Mechanisms of nanoparticle-induced oxidative stress and toxicity. Biomed Res. Int. 2013:942916
Marizcurrena JJ, Acosta S, Canclini L et al (2020) A natural occurring bifunctional CPD/(6–4)-photolyase from the Antarctic bacterium Sphingomonas sp. UV9. Appl Microbiol Biotechnol 104:7037–7050. https://doi.org/10.1007/s00253-020-10734-5
Market Research Report (2019) Natural and organic cosmetics market size, share, segmentation by type (skin care, hair care, oral care, makeup cosmetics and others), by consumer group (male and female), by distribution channel (store-based and non-store based) – global forecast till 20
Market Research Report (2021) The global cosmetic products market by product type
Martins A, Vieira H, Gaspar H, Santos S (2014a) Marketed marine natural products in the pharmaceutical and cosmeceutical industries: tips for success. Mar Drugs 12:1066–1101
Martins IM, Barreiro MF, Coelho M, Rodrigues AE (2014b) Microencapsulation of essential oils with biodegradable polymeric carriers for cosmetic applications. Chem Eng J 245:191–200
Matta MK, Zusterzeel R, Pilli NR et al (2019) Effect of sunscreen application under maximal use conditions on plasma concentration of sunscreen active ingredients: a randomized clinical trial. JAMA - J Am Med Assoc 321:2082–2091. https://doi.org/10.1001/jama.2019.5586
Matwiejczuk N, Galicka A, Brzóska MM (2020) Review of the safety of application of cosmetic products containing parabens. J Appl Toxicol 40:176–210
McPhee D, Pin A, Kizer L, Perelman L (2014) Squalane from Sugarcane. Cosmet Toilet Mag 129:1–6
Mellage C (2016) Cosmetic textiles – where beauty meets fashion. In: Kline Co. https://www.klinegroup.com/cosmetic-textiles-where-beauty-meets-fashion/
Miastkowska M, Sikora E (2018) Anti-aging properties of plant stem cell extracts. Cosmetics 5:55
Mihranyan A, Ferraz N, Strømme M (2012) Current status and future prospects of nanotechnology in cosmetics. Prog Mater Sci 57:875–910
Miller N (2020) How factories change production to quicky fight coronavirus. Bbc 1–5
Miranda MS, Cintra RG, Barros SBM, Mancini-Filho J (1998) Antioxidant activity of the microalga Spirulina maxima. Brazilian J Med Biol Res 31:1075–1079. https://doi.org/10.1590/S0100-879X1998000800007
Mitura S, Sionkowska A, Jaiswal A (2020) Biopolymers for hydrogels in cosmetics: review. J Mater Sci Mater Med 31:1–14
Morais FP, Simões RMS, Curto JMR (2020) Biopolymeric delivery systems for cosmetic applications using chlorella vulgaris algae and tea tree essential oil. Polymers (basel) 12:1–13. https://doi.org/10.3390/polym12112689
Murariu M, Dubois P (2016) PLA composites: from production to properties. Adv Drug Deliv Rev 107:17–46
Nainggolan M, Sinaga AGS (2021) The modification of red palm oil and palm kernel oil as antibacterial liquid soap. Rasayan J Chem 14:36–40. https://doi.org/10.31788/RJC.2021.1415848
Nandy A, Lee E, Mandal A et al (2020) Microencapsulation of retinyl palmitate by melt dispersion for cosmetic application. J Microencapsul 37:205–219. https://doi.org/10.1080/02652048.2020.1720029
Nardelli A, Drieghe J, Claes L et al (2011) Fragrance allergens in “specific” cosmetic products. Contact Dermatitis 64:212–219. https://doi.org/10.1111/j.1600-0536.2011.01877.x
Naveed Ul Haq A, Nadhman A, Ullah I et al (2017) Synthesis approaches of zinc oxide nanoparticles: the dilemma of ecotoxicity. J. Nanomater. 2017:1–14
Novak AC, Sydney EB, Soccol CR (2014) Biocosmetics. In: Biotransformation of Waste Biomass into High Value Biochemicals. pp 389–411
Ó briain O, Marques Mendes AR, McCarron S et al (2020) The role of wet wipes and sanitary towels as a source of white microplastic fibres in the marine environment. Water Res 182:116021. https://doi.org/10.1016/j.watres.2020.116021
Oberdörster G, Oberdörster E, Oberdörster J (2005) Nanotoxicology: an emerging discipline evolving from studies of ultrafine particles. Environ Health Perspect 113:823–839
Oberdörster G, Sharp Z, Atudorei V et al (2002) Extrapulmonary translocation of ultrafine carbon particles following whole-body inhalation exposure of rats. J Toxicol Environ Heal - Part A 65:1531–1543. https://doi.org/10.1080/00984100290071658
Oh HJ, Kim J (2020) Characterization of inhalable aerosols from cosmetic powders and sustainability in cosmetic products. Sustain 12:8187. https://doi.org/10.3390/su12198187
Ohgitani E, Shin-Ya M, Ichitani M et al (2021) Rapid inactivation in vitro of sars-cov-2 in saliva by black tea and green tea. Pathogens 10:721
Osowska N, Ruzik L (2019) New potentials in the extraction of trace metal using natural deep eutectic solvents (NADES). Food Anal Methods 12:926–935. https://doi.org/10.1007/s12161-018-01426-y
Oya Y, Yoshida H, Takeshima M et al (2000) Beneficial effect of eyelid make-up (natural rubber latex) to induce a new fold in the treatment of blepharoptosis in myotonic dystrophy. Clin Neurol 40:483–486
Pacifico S, Piccolella S, Veneziano R New sustainable cosmetic products from food waste: a joined-up approach between design and food chemistry. In: The learning network on sustainabiity. Italy
Paiva-Santos AC, Herdade AM, Guerra C, et al (2021) Plant-mediated green synthesis of metal-based nanoparticles for dermopharmaceutical and cosmetic applications. Int. J. Pharm. 597
Pan NC, Vignoli JA, Baldo C et al (2015) Effect of fermentation conditions on the production of hyaluronic acid by streptococcus zooepidemicus ATCC 39920. Acta Sci - Biol Sci 37:411–417. https://doi.org/10.4025/actascibiolsci.v37i4.28176
Panico A, Serio F, Bagordo F et al (2019) Skin safety and health prevention: an overview of chemicals in cosmetic products. J Prev Med Hyg 60:50–57. https://doi.org/10.15167/2421-4248/jpmh2019.60.1.1080
Park EJ, Garcia CV, Youn SJ et al (2019) Fortification of γ-aminobutyric acid and bioactive compounds in Cucurbita moschata by novel two-step fermentation using Bacillus subtilis and Lactobacillus plantarum. Lwt 102:22–29. https://doi.org/10.1016/j.lwt.2018.07.065
Patil AS, Patil AV, Patil AH et al (2017) A review on: standerdization of herb in new era of cosmaceuticals: herbal cosmetics. Patil Al World J Pharm Res 6:303
Patravale VB, Mandawgade SD (2008) Novel cosmetic delivery systems: an application update. Int J Cosmet Sci 30:19–33
Perioli L, Ambrogi V, Rossi C et al (2006) Use of anionic clays for photoprotection and sunscreen photostability: hydrotalcites and phenylbenzimidazole sulfonic acid. J Phys Chem Solids 67:1079–1083. https://doi.org/10.1016/j.jpcs.2006.01.029
Perioli L, Nocchetti M, Ambrogi V et al (2008) Sunscreen immobilization on ZnAl-hydrotalcite for new cosmetic formulations. Microporous Mesoporous Mater 107:180–189. https://doi.org/10.1016/j.micromeso.2007.02.021
Phua G (2020) Understanding the cosmetic regulations in australia. In: Formula Bot. https://formulabotanica.com/cosmetic-regulations-in-australia/
Pichayakorn W, Boonme P, Taweepreda W (2013) Preparation of peel-off mask from deproteinized natural rubber latex. In: Advanced Materials Research. pp 95–98
Pistollato F, Madia F, Corvi R et al (2021) Current EU regulatory requirements for the assessment of chemicals and cosmetic products: challenges and opportunities for introducing new approach methodologies. Arch Toxicol 95:1867–1897. https://doi.org/10.1007/s00204-021-03034-y
Prakalathan K, Mohanty S, Nayak SK (2012) Polylactide/modified layered silicates nanocomposites: a critical analysis of morphological, mechanical and thermal properties. J Reinf Plast Compos 31:1300–1310. https://doi.org/10.1177/0731684412457597
R. Sahu R, P. Saxena SJ (2014) Heavy metals in Cosmetics. J Sci Environent 101:1–28
Rahnamaeian M, Vilcinskas A (2015) Short antimicrobial peptides as cosmetic ingredients to deter dermatological pathogens. Appl Microbiol Biotechnol 99:8847–8855
Raj RK, Chandrul KK (2016) Regulatory requirements for cosmetics in relation with regulatory authorities in India against US, Europe, Australia and Asean Countries. Int J Pharma Res Heal Sci 4:1332–41. https://doi.org/10.21276/ijprhs.2016.05.01
Raj S, Jose S, Sumod US, Sabitha M (2012) Nanotechnology in cosmetics: opportunities and challenges. J Pharm Bioallied Sci 4:186–193
Rana ASMRASK (2021) Hand sanitizer with natural ingredients exhibits enhanced antimicrobial efficacy
Ribeiro HL, de Oliveira AV, Brito ES, d, et al (2018) Stabilizing effect of montmorillonite on acerola juice anthocyanins. Food Chem 245:966–973. https://doi.org/10.1016/j.foodchem.2017.11.076
Rinaldi A (2008) Healing beauty? More biotechnology cosmetic products that claim drug-like properties reach the market. EMBO Rep 9:1073–1077. https://doi.org/10.1038/embor.2008.200
Rose PM, Cantrill V, Benohoud M et al (2018) Application of anthocyanins from blackcurrant (Ribes nigrum L.) Fruit Waste as Renewable Hair Dyes. J Agric Food Chem 66:6790–6798. https://doi.org/10.1021/acs.jafc.8b01044
Rovani S, Santos JJ, Corio P, Fungaro DA (2018) Highly pure silica nanoparticles with high adsorption capacity obtained from sugarcane waste ash. ACS Omega 3:2618–2627. https://doi.org/10.1021/acsomega.8b00092
Ryu SJ, Jung H, Oh JM et al (2010) Layered double hydroxide as novel antibacterial drug delivery system. J Phys Chem Solids 71:685–688. https://doi.org/10.1016/j.jpcs.2009.12.066
S. Babitha EK (2011) Prospects and trends of marine-derived cosmeceutical ingredients. In: Marine cosmeceuticals: trends and prospects. pp 63–65
Sajna KV, Gottumukkala LD, Sukumaran RK, Pandey A (2015) White biotechnology in cosmetics. In: Industrial Biorefineries and White Biotechnology. pp 607–652
Santos-Buelga C, González-Paramás AM (2018) Anthocyanins. In: Encyclopedia of Food Chemistry. pp 10–21
Saxena R, Mittal P, Clavaud C et al (2018) Comparison of healthy and dandruff scalp microbiome reveals the role of commensals in scalp health. Front Cell Infect Microbiol 8:346. https://doi.org/10.3389/fcimb.2018.00346
SCCS, Rousselle C (2015) Opinion of the scientific committee on consumer safety (SCCS) - revision of the opinion on the safety of aluminium in cosmetic products
Secchi M, Castellani V, Collina E et al (2016) Assessing eco-innovations in green chemistry: life cycle assessment (LCA) of a cosmetic product with a bio-based ingredient. J Clean Prod 129:269–281. https://doi.org/10.1016/j.jclepro.2016.04.073
Sienkiewicz M, Denys P, Kowalczyk E (2011) Antibacterial and immunostimulatory effect of essential oils. Int Rev Allergol Clin Immunol 17:40–44
Smith HR, Wakelin SH, White IR (1998) Banana hair conditioner and natural rubber latex allergy. Contact Dermatitis 39:202. https://doi.org/10.1111/j.1600-0536.1998.tb05901.x
Soares M, Vitorino C, Sousa J, Pais A (2015) Skin permeation: challenges and opportunities. Rev Ciencias Farm Basica e Apl 21:2698–2712
Steinemann A, Nematollahi N, Rismanchi B et al (2021) Pandemic products and volatile chemical emissions. Air Qual Atmos Heal 14:47–53. https://doi.org/10.1007/s11869-020-00912-9
Stiger-Pouvreau V, Guerard F (2018) Bio-inspired molecules extracted from marine macroalgae: a new generation of active ingredients for cosmetics and human health. In: Blue Biotechnology. pp 709–746
Subhashini V, Bhojraj S, Keshav Prakash S, Shalilni T (2017) A complexity focus on nanotoxicology- a review. Res J Pharm Technol 10:346–350
Sumaiyah A, Leisyah BM (2019) The effect of antioxidant of grapeseed oil as skin anti-aging in nanoemulsion and emulsion preparations. Rasayan J Chem 12:1185–1194. https://doi.org/10.31788/RJC.2019.1235337
Abdullah TSH, Mohd AR, Asseri SN, Mohamad KW et al (2021) Green synthesis, characterization and applications of silver nanoparticle mediated by the aqueous extract of red onion peel. Environ Pollut 271:116295. https://doi.org/10.1016/j.envpol.2020.116295
Thiyagarasaiyar K, Goh BH, Jeon YJ, Yow YY (2020) Algae metabolites in cosmeceutical: an overview of current applications and challenges. Mar. Drugs 18:323
Toschi TG, Cardenia V, Bonaga G et al (2014) Coffee silverskin: characterization, possible uses, and safety aspects. J Agric Food Chem 62:10836–10844. https://doi.org/10.1021/jf503200z
Vandamme E (2001) Biocosmetics produced via microbial and enzymatic synthesis. Agro Food Ind Hi Tech 12:11–18
Vandenberghe LPS, Karp SG, de Oliveira PZ, et al (2018) Solid-state fermentation for the production of organic acids. In: Current Developments in Biotechnology and Bioengineering. pp 415–434
Velasquillo C, Galue EA, Rodriquez L et al (2013) Skin 3D bioprinting. Applications in Cosmetology. J Cosmet Dermatological Sci Appl 03:85–89. https://doi.org/10.4236/jcdsa.2013.31a012
Vinet L, Zhedanov A (2011) A “missing” family of classical orthogonal polynomials. J Phys A Math Theor 44:22–23. https://doi.org/10.1088/1751-8113/44/8/085201
Wang J, Liu Y, Kam WR et al (2020) Toxicity of the cosmetic preservatives parabens, phenoxyethanol and chlorphenesin on human meibomian gland epithelial cells. Exp Eye Res 196:108057. https://doi.org/10.1016/j.exer.2020.108057
Wawrzynczak A, Feliczak-Guzik A, Nowak I (2016) Nanosunscreens: from nanoencapsulated to nanosized cosmetic active forms. In: Nanobiomaterials in Galenic Formulations and Cosmetics: Applications of Nanobiomaterials. pp 25–46
Weidmann J, Craik DJ (2016) Discovery, structure, function, and applications of cyclotides: circular proteins from plants. J Exp Bot 67:4801–4812
Weinberg WC, Goodman LV, George C et al (1993) Reconstitution of hair follicle development in vivo: determination of follicle formation, hair growth, and hair quality by dermal cells. J Invest Dermatol 100:229–236. https://doi.org/10.1111/1523-1747.ep12468971
Wood L (2020) Impact of COVID-19 on the global cosmetic industry. ResearchAndMarkets 1–6
Wu J-L, Lu J-K (2011) Aquatic and marine bioactive antimicrobial peptides and biosurfactants for the cosmeceutical industry. In: Marine Cosmeceuticals. pp 371–390
Xu X, Wu B, Zhao W et al (2021) Shifts in autochthonous microbial diversity and volatile metabolites during the fermentation of chili pepper (Capsicum frutescens L.). Food Chem 335:127512. https://doi.org/10.1016/j.foodchem.2020.127512
Yan WC, Davoodi P, Vijayavenkataraman S et al (2018) 3D bioprinting of skin tissue: from pre-processing to final product evaluation. Adv Drug Deliv Rev 132:270–295
Zarei F, Abbaszadeh A (2018) Stem cell and skin rejuvenation. J Cosmet Laser Ther 20:193–197
Zaza S, Reeder JM, Charles LE, Jarvis WR (1994) Latex sensitivity among perioperative nurses. AORN J 60:806–812. https://doi.org/10.1016/S0001-2092(07)63329-8
Zhong X, Downs CA, Li Y et al (2020) Comparison of toxicological effects of oxybenzone, avobenzone, octocrylene, and octinoxate sunscreen ingredients on cucumber plants (Cucumis sativus L.). Sci Total Environ 714:136879. https://doi.org/10.1016/j.scitotenv.2020.136879
Download references
Acknowledgements
I thank the University of Petroleum and Energy Studies for supporting this research. I also thank Prof. Neeraj Mahindroo and Prof. Manish Gupta, School of Health Sciences at University of Petroleum and Energy Studies, and anonymous referees for their useful suggestions in the improvement of this work.
Author information
Authors and affiliations.
Department of Chemical Engineering, University of Petroleum and Energy Studies, Dehradun, 248007, India
Nishu Goyal & Frankline Jerold
You can also search for this author in PubMed Google Scholar
Contributions
NG drafted the work, revised it critically, and submitted the manuscript. FJ collected the tabular data.
Corresponding author
Correspondence to Nishu Goyal .
Ethics declarations
Ethics approval, consent to participate.
The authors read and approved the final manuscript.
Consent for publication
Conflict of interest.
The authors declare no competing interests.
Additional information
Responsible Editor: Philippe Garrigues
Publisher's Note
Springer Nature remains neutral with regard to jurisdictional claims in published maps and institutional affiliations.
Rights and permissions
Reprints and permissions
About this article
Goyal, N., Jerold, F. Biocosmetics: technological advances and future outlook. Environ Sci Pollut Res 30 , 25148–25169 (2023). https://doi.org/10.1007/s11356-021-17567-3
Download citation
Received : 01 June 2021
Accepted : 12 November 2021
Published : 25 November 2021
Issue Date : February 2023
DOI : https://doi.org/10.1007/s11356-021-17567-3
Share this article
Anyone you share the following link with will be able to read this content:
Sorry, a shareable link is not currently available for this article.
Provided by the Springer Nature SharedIt content-sharing initiative
- Ingredients
- Formulations
- Find a journal
- Publish with us
- Track your research

Cosmetics News
Top headlines, latest headlines.
- Why Skin Gets 'Leathery' After Too Much Sun
- Potential Multiple Health Benefits During Aging
- Anti-Aging Potential in an Invasive Weed
- Laser Treatments to Prevent Skin Cancer
- UV-Emitting Nail Polish Dryers: Health Concern?
- Scars Mended Using Transplanted Hair Follicles
- Painless Tattoos That Can Be Self-Administered
- Nanodiamonds: Delivering Remedies Through Skin
- Better Acne Scar Treatments
- Other People Don't Really Notice Facial Scars
Earlier Headlines
Wednesday, january 26, 2022.
- New Hair Dyes Avoid Allergic Reactions
Wednesday, January 5, 2022
- Can We Go from Scarface to Scarless?
Thursday, August 5, 2021
- New Drug Molecules Could Prevent Skin Aging Caused by Sun Exposure
Tuesday, June 8, 2021
- Internal Compression Stocking Helps Against Varicose Veins
- LATEST NEWS
- Health & Medicine
- Diseases & Conditions
- Alzheimer's Research
- Amyotrophic Lateral Sclerosis
- Attention Deficit Disorder
- Back and Neck Pain
- Birth Defects
- Bladder Disorders
- Blood Clots
- COVID and SARS
- Cervical Cancer
- Bladder Cancer
- Multiple Myeloma
- Pancreatic Cancer
- Brain Tumor
- Colon Cancer
- Breast Cancer
- Ovarian Cancer
- Lung Cancer
- Mesothelioma
- Skin Cancer
- Prostate Cancer
- Cerebral Palsy
- Chikungunya
- Chronic Fatigue Syndrome
- Cold and Flu
- Crohn's Disease
- Cystic Fibrosis
- Dengue Fever
- Down Syndrome
- Eating Disorder Research
- Encephalitis
- Epilepsy Research
- Erectile Dysfunction
- Fibromyalgia
- Gastrointestinal Problems
- HIV and AIDS
- Headache Research
- Hearing Loss
- Heart Health
- Cholesterol
- Stroke Prevention
- Heart Disease
- Hormone Disorders
- Hypertension
- Infectious Diseases
- Insomnia Research
- Irritable Bowel Syndrome
- Kidney Disease
- Liver Disease
- Lung Disease
- Lyme Disease
- Mental Health Research
- Multiple Sclerosis Research
- Mumps, Measles, Rubella
- Muscular Dystrophy
- Osteoporosis
- Parkinson's Research
- Prostate Health
- Restless Leg Syndrome
- Sickle Cell Anemia
- Sleep Disorder Research
- Thyroid Disease
- Triglycerides
- Tuberculosis
- Medical Topics
- Accident and Trauma
- Alternative Medicine
- Birth Control
- Bone and Spine
- Chronic Illness
- Controlled Substances
- Dietary Supplements and Minerals
- Epigenetics
- Food Additives
- Foodborne Illness
- Foot Health
- Gene Therapy
- Health Policy
- Human Biology
- Immune System
- Joint Health
- Medical Imaging
- Nervous System
- Pain Control
- Personalized Medicine
- Pharmacology
- Psychology Research
- Wounds and Healing
- PHYSICAL/TECH
- ENVIRONMENT
- SOCIETY & EDUCATION
- Universal, Long-Lasting Flu Shot
- Better Vision from CRISPR Gene Editing Trial
- Why Venus Has Almost No Water
- Stellar Light Surrounding Ancient Quasars
- New Discoveries About Jupiter
- Simulations Support Dark Matter Theory
- 3D Printed Programmable Living Materials
- Emergence of Animals: Magnetic Field Collapse
- Ice Shelves Crack from Weight of Meltwater Lakes
- Countries' Plans to Remove CO2 Not Enough
Trending Topics
Strange & offbeat.
- Research article
- Open access
- Published: 12 June 2019
The impact of skin care products on skin chemistry and microbiome dynamics
- Amina Bouslimani 1 na1 ,
- Ricardo da Silva 1 na1 ,
- Tomasz Kosciolek 2 ,
- Stefan Janssen 2 , 3 ,
- Chris Callewaert 2 , 4 ,
- Amnon Amir 2 ,
- Kathleen Dorrestein 1 ,
- Alexey V. Melnik 1 ,
- Livia S. Zaramela 2 ,
- Ji-Nu Kim 2 ,
- Gregory Humphrey 2 ,
- Tara Schwartz 2 ,
- Karenina Sanders 2 ,
- Caitriona Brennan 2 ,
- Tal Luzzatto-Knaan 1 ,
- Gail Ackermann 2 ,
- Daniel McDonald 2 ,
- Karsten Zengler 2 , 5 , 6 ,
- Rob Knight 2 , 5 , 6 , 7 &
- Pieter C. Dorrestein 1 , 2 , 5 , 8
BMC Biology volume 17 , Article number: 47 ( 2019 ) Cite this article
97k Accesses
92 Citations
123 Altmetric
Metrics details
Use of skin personal care products on a regular basis is nearly ubiquitous, but their effects on molecular and microbial diversity of the skin are unknown. We evaluated the impact of four beauty products (a facial lotion, a moisturizer, a foot powder, and a deodorant) on 11 volunteers over 9 weeks.
Mass spectrometry and 16S rRNA inventories of the skin revealed decreases in chemical as well as in bacterial and archaeal diversity on halting deodorant use. Specific compounds from beauty products used before the study remain detectable with half-lives of 0.5–1.9 weeks. The deodorant and foot powder increased molecular, bacterial, and archaeal diversity, while arm and face lotions had little effect on bacterial and archaeal but increased chemical diversity. Personal care product effects last for weeks and produce highly individualized responses, including alterations in steroid and pheromone levels and in bacterial and archaeal ecosystem structure and dynamics.
Conclusions
These findings may lead to next-generation precision beauty products and therapies for skin disorders.
The human skin is the most exposed organ to the external environment and represents the first line of defense against external chemical and microbial threats. It harbors a microbial habitat that is person-specific and varies considerably across the body surface [ 1 , 2 , 3 , 4 ]. Recent findings suggested an association between the use of antiperspirants or make-up and skin microbiota composition [ 5 , 6 , 7 ]. However, these studies were performed for a short period (7–10 days) and/or without washing out the volunteers original personal care products, leading to incomplete evaluation of microbial alterations because the process of skin turnover takes 21–28 days [ 5 , 6 , 7 , 8 , 9 ]. It is well-established that without intervention, most adult human microbiomes, skin or other microbiomes, remain stable compared to the differences between individuals [ 3 , 10 , 11 , 12 , 13 , 14 , 15 , 16 ].
Although the skin microbiome is stable for years [ 10 ], little is known about the molecules that reside on the skin surface or how skin care products influence this chemistry [ 17 , 18 ]. Mass spectrometry can be used to detect host molecules, personalized lifestyles including diet, medications, and personal care products [ 18 , 19 ]. However, although the impact of short-term dietary interventions on the gut microbiome has been assessed [ 20 , 21 ], no study has yet tested how susceptible the skin chemistry and Microbiome are to alterations in the subjects’ personal care product routine.
In our recent metabolomic/microbiome 3D cartography study [ 18 ], we observed altered microbial communities where specific skin care products were present. Therefore, we hypothesized that these products might shape specific skin microbial communities by changing their chemical environment. Some beauty product ingredients likely promote or inhibit the growth of specific bacteria: for example, lipid components of moisturizers could provide nutrients and promote the growth of lipophilic bacteria such as Staphylococcus and Propionibacterium [ 18 , 22 , 23 ]. Understanding both temporal variations of the skin microbiome and chemistry is crucial for testing whether alterations in personal habits can influence the human skin ecosystem and, perhaps, host health. To evaluate these variations, we used a multi-omics approach integrating metabolomics and microbiome data from skin samples of 11 healthy human individuals. Here, we show that many compounds from beauty products persist on the skin for weeks following their use, suggesting a long-term contribution to the chemical environment where skin microbes live. Metabolomics analysis reveals temporal trends correlated to discontinuing and resuming the use of beauty products and characteristic of variations in molecular composition of the skin. Although highly personalized, as seen with the microbiome, the chemistry, including hormones and pheromones such as androstenone and androsterone, were dramatically altered. Similarly, by experimentally manipulating the personal care regime of participants, bacterial and molecular diversity and structure are altered, particularly for the armpits and feet. Interestingly, a high person-to-person molecular and bacterial variability is maintained over time even though personal care regimes were modified in exactly the same way for all participants.
Skin care and hygiene products persist on the skin
Systematic strategies to influence both the skin chemistry and microbiome have not yet been investigated. The outermost layer of the skin turns over every 3 to 4 weeks [ 8 , 9 ]. How the microbiome and chemistry are influenced by altering personal care and how long the chemicals of personal care products persist on the skin are essentially uncharacterized. In this study, we collected samples from skin of 12 healthy individuals—six males and six females—over 9 weeks. One female volunteer had withdrawn due to skin irritations that developed, and therefore, we describe the remaining 11 volunteers. Samples were collected from each arm, armpit, foot, and face, including both the right and left sides of the body (Fig. 1 a). All participants were asked to adhere to the same daily personal care routine during the first 6 weeks of this study (Fig. 1 b). The volunteers were asked to refrain from using any personal care product for weeks 1–3 except a mild body wash (Fig. 1 b). During weeks 4–6, in addition to the body wash, participants were asked to apply selected commercial skin care products at specific body parts: a moisturizer on the arm, a sunscreen on the face, an antiperspirant on the armpits, and a soothing powder on the foot (Fig. 1 b). To monitor adherence of participants to the study protocol, molecular features found in the antiperspirant, facial lotion, moisturizer, and foot powder were directly tracked with mass spectrometry from the skin samples. For all participants, the mass spectrometry data revealed the accumulation of specific beauty product ingredients during weeks 4–6 (Additional file 1 : Figure S1A-I, Fig. 2 a orange arrows). Examples of compounds that were highly abundant during T4–T6 in skin samples are avobenzone (Additional file 1 : Figure S1A), dexpanthenol (Additional file 1 : Figure S1B), and benzalkonium chloride (Additional file 1 : Figure S1C) from the facial sunscreen; trehalose 6-phosphate (Additional file 1 : Figure S1D) and glycerol stearate (Additional file 1 : Figure S1E) from the moisturizer applied on arms; indolin (Additional file 1 : Figure S1F) and an unannotated compound ( m/z 233.9, rt 183.29 s) (Additional file 1 : Figure S1G) from the foot powder; and decapropylene glycol (Additional file 1 : Figure S1H) and nonapropylene glycol (Additional file 1 : Figure S1I) from the antiperspirant. These results suggest that there is likely a compliance of all individuals to study requirements and even if all participants confirmed using each product every day, the amount of product applied by each individual may vary. Finally, for weeks 7–9, the participants were asked to return to their normal routine by using the same personal care products they used prior to the study. In total, excluding all blanks and personal care products themselves, we analyzed 2192 skin samples for both metabolomics and microbiome analyses.
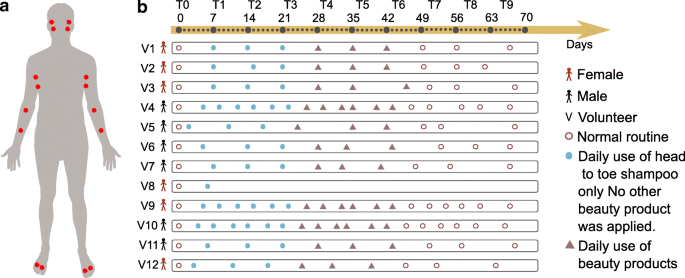
Study design and representation of changes in personal care regime over the course of 9 weeks. a Six males and six females were recruited and sampled using swabs on two locations from each body part (face, armpits, front forearms, and between toes) on the right and left side. The locations sampled were the face—upper cheek bone and lower jaw, armpit—upper and lower area, arm—front of elbow (antecubitis) and forearm (antebrachium), and feet—in between the first and second toe and third and fourth toe. Volunteers were asked to follow specific instructions for the use of skin care products. b Following the use of their personal skin care products (brown circles), all volunteers used only the same head to toe shampoo during the first 3 weeks (week 1–week 3) and no other beauty product was applied (solid blue circle). The following 3 weeks (week 4–week 6), four selected commercial beauty products were applied daily by all volunteers on the specific body part (deodorant antiperspirant for the armpits, soothing foot powder for the feet between toes, sunscreen for the face, and moisturizer for the front forearm) (triangles) and continued to use the same shampoo. During the last 3 weeks (week 7–week 9), all volunteers went back to their normal routine and used their personal beauty products (circles). Samples were collected once a week (from day 0 to day 68—10 timepoints from T0 to T9) for volunteers 1, 2, 3, 4, 5, 6, 7, 9, 10, 11, and 12, and on day 0 and day 6 for volunteer 8, who withdraw from the study after day 6. For 3 individuals (volunteers 4, 9, 10), samples were collected twice a week (19 timepoints total). Samples collected for 11 volunteers during 10 timepoints: 11 volunteers × 10 timepoints × 4 samples × 4 body sites = 1760. Samples collected from 3 selected volunteers during 9 additional timepoints: 3 volunteers × 9 timepoints × 4 samples × 4 body sites = 432. See also the “ Subject recruitment and sample collection ” section in the “ Methods ” section
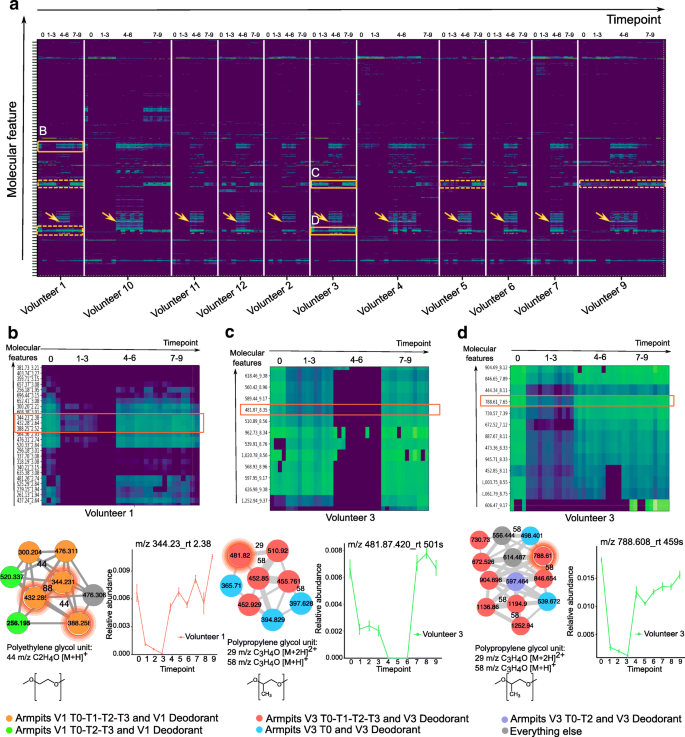
Monitoring the persistence of personal care product ingredients in the armpits over a 9-week period. a Heatmap representation of the most abundant molecular features detected in the armpits of all individuals during the four phases (0: initial, 1–3: no beauty products, 4–6: common products, and 7–9: personal products). Green color in the heatmap represents the highest molecular abundance and blue color the lowest one. Orange boxes with plain lines represent enlargement of cluster of molecules that persist on the armpits of volunteer 1 ( b ) and volunteer 3 ( c , d ). Orange clusters with dotted lines represent same clusters of molecules found on the armpits of other volunteers. Orange arrows represent the cluster of compounds characteristic of the antiperspirant used during T4–T6. b Polyethylene glycol (PEG) molecular clusters that persist on the armpits of individual 1. The molecular subnetwork, representing molecular families [ 24 ], is part of a molecular network ( http://gnps.ucsd.edu/ProteoSAFe/status.jsp?task=f5325c3b278a46b29e8860ec5791d5ad ) generated from MS/MS data collected from the armpits of volunteer 1 (T0–T3) MSV000081582 and MS/MS data collected from the deodorant used by volunteer 1 before the study started (T0) MSV000081580. c , d Polypropylene glycol (PPG) molecular families that persist on the armpits of individual 3, along with the corresponding molecular subnetwork that is part of the molecular network accessible here http://gnps.ucsd.edu/ProteoSAFe/status.jsp?task=aaa1af68099d4c1a87e9a09f398fe253 . Subnetworks were generated from MS/MS data collected from the armpits of volunteer 3 (T0–T3) MSV000081582 and MS/MS data collected from the deodorant used by volunteer 3 at T0 MSV000081580. The network nodes were annotated with colors. Nodes represent MS/MS spectra found in armpit samples of individual 1 collected during T0, T1, T2, and T3 and in personal deodorant used by individual 1 (orange nodes); armpit samples of individual 1 collected during T0, T2, and T3 and personal deodorant used by individual 1 (green nodes); armpit samples of individual 3 collected during T0, T1, T2, and T3 and in personal deodorant used by individual 3 (red nodes); armpit samples of individual 3 collected during T0 and in personal deodorant used by individual 3 (blue nodes); and armpit samples of individual 3 collected during T0 and T2 and in personal deodorant used by individual 3 (purple nodes). Gray nodes represent everything else. Error bars represent standard error of the mean calculated at each timepoint from four armpit samples collected from the right and left side of each individual separately. See also Additional file 1 : Figure S1
To understand how long beauty products persist on the skin, we monitored compounds found in deodorants used by two volunteers—female 1 and female 3—before the study (T0), over the first 3 weeks (T1–T3) (Fig. 1 b). During this phase, all participants used exclusively the same body wash during showering, making it easier to track ingredients of their personal care products. The data in the first 3 weeks (T1–T3) revealed that many ingredients of deodorants used on armpits (Fig. 2 a) persist on the skin during this time and were still detected during the first 3 weeks or at least during the first week following the last day of use. Each of the compounds detected in the armpits of individuals exhibited its own unique half-life. For example, the polyethylene glycol (PEG)-derived compounds m/z 344.227, rt 143 s (Fig. 2 b, S1J); m/z 432.279, rt 158 s (Fig. 2 b, S1K); and m/z 388.253, rt 151 s (Fig. 2 b, S1L) detected on armpits of volunteer 1 have a calculated half-life of 0.5 weeks (Additional file 1 : Figure S1J-L, all p values < 1.81e−07), while polypropylene glycol (PPG)-derived molecules m/z 481.87, rt 501 s (Fig. 2 c, S1M); m/z 560.420, rt 538 s (Fig. 2 c, S1N); m/z 788.608, rt 459 s (Fig. 2 d, S1O); m/z 846.650, rt 473 s (Fig. 2 d, S1P); and m/z 444.338, rt 486 s (Fig. 2 d, S1Q) found on armpits of volunteers 3 and 1 (Fig. 2 a) have a calculated half-life ranging from 0.7 to 1.9 weeks (Additional file 1 : Figure S1M-Q, all p values < 0.02), even though they originate from the same deodorant used by each individual. For some ingredients of deodorant used by volunteer 3 on time 0 (Additional file 1 : Figure S1M, N), a decline was observed during the first week, then little to no traces of these ingredients were detected during weeks 4–6 (T4–T6), then finally these ingredients reappear again during the last 3 weeks of personal product use (T7–T9). This suggests that these ingredients are present exclusively in the personal deodorant used by volunteer 3 before the study. Because a similar deodorant (Additional file 1 : Figure S1O-Q) and a face lotion (Additional file 1 : Figure S1R) was used by volunteer 3 and volunteer 2, respectively, prior to the study, there was no decline or absence of their ingredients during weeks 4–6 (T4–T6).
Polyethylene glycol compounds (Additional file 1 : Figure S1J-L) wash out faster from the skin than polypropylene glycol (Additional file 1 : Figure S1M-Q)(HL ~ 0.5 weeks vs ~ 1.9 weeks) and faster than fatty acids used in lotions (HL ~ 1.2 weeks) (Additional file 1 : Figure S1R), consistent with their hydrophilic (PEG) and hydrophobic properties (PPG and fatty acids) [ 25 , 26 ]. This difference in hydrophobicity is also reflected in the retention time as detected by mass spectrometry. Following the linear decrease of two PPG compounds from T0 to T1, they accumulated noticeably during weeks 2 and 3 (Additional file 1 : Figure S1M, N). This accumulation might be due to other sources of PPG such as the body wash used during this period or the clothes worn by person 3. Although PPG compounds were not listed in the ingredient list of the shampoo, we manually inspected the LC-MS data collected from this product and confirmed the absence of PPG compounds in the shampoo. The data suggest that this trend is characteristic of accumulation of PPG from additional sources. These could be clothes, beds, or sheets, in agreement with the observation of these molecules found in human habitats [ 27 ] but also in the public GNPS mass spectrometry dataset MSV000079274 that investigated the chemicals from dust collected from 1053 mattresses of children.
Temporal molecular and bacterial diversity in response to personal care use
To assess the effect of discontinuing and resuming the use of skin care products on molecular and microbiota dynamics, we first evaluated their temporal diversity. Skin sites varied markedly in their initial level (T0) of molecular and bacterial diversity, with higher molecular diversity at all sites for female participants compared to males (Fig. 3 a, b, Wilcoxon rank-sum-WR test, p values ranging from 0.01 to 0.0001, from foot to arm) and higher bacterial diversity in face (WR test, p = 0.0009) and armpits (WR test, p = 0.002) for females (Fig. 3 c, d). Temporal diversity was similar across the right and left sides of each body site of all individuals (WR test, molecular diversity: all p values > 0.05; bacterial diversity: all p values > 0.20). The data show that refraining from using beauty products (T1–T3) leads to a significant decrease in molecular diversity at all sites (Fig. 3 a, b, WR test, face: p = 8.29e−07, arm: p = 7.08e−09, armpit: p = 1.13e−05, foot: p = 0.002) and bacterial diversity mainly in armpits (WR test, p = 0.03) and feet (WR test, p = 0.04) (Fig. 3 c, d). While molecular diversity declined (Fig. 3 a, b) for arms and face, bacterial diversity (Fig. 3 c, d) was less affected in the face and arms when participants did not use skin care products (T1–T3). The molecular diversity remained stable in the arms and face of female participants during common beauty products use (T4–T6) to immediately increase as soon as the volunteers went back to their normal routines (T7–T9) (WR test, p = 0.006 for the arms and face)(Fig. 3 a, b). A higher molecular (Additional file 1 : Figure S2A) and community (Additional file 1 : Figure S2B) diversity was observed for armpits and feet of all individuals during the use of antiperspirant and foot powder (T4–T6) (WR test, molecular diversity: armpit p = 8.9e−33, foot p = 1.03e−11; bacterial diversity: armpit p = 2.14e−28, foot p = 1.26e−11), followed by a molecular and bacterial diversity decrease in the armpits when their regular personal beauty product use was resumed (T7–T9) (bacterial diversity: WR test, p = 4.780e−21, molecular diversity: WR test, p = 2.159e−21). Overall, our data show that refraining from using beauty products leads to lower molecular and bacterial diversity, while resuming the use increases their diversity. Distinct variations between male and female molecular and community richness were perceived at distinct body parts (Fig. 3 a–d). Although the chemical diversity of personal beauty products does not explain these variations (Additional file 1 : Figure S2C), differences observed between males and females may be attributed to many environmental and lifestyle factors including different original skin care and different frequency of use of beauty products (Additional file 2 : Table S1), washing routines, and diet.
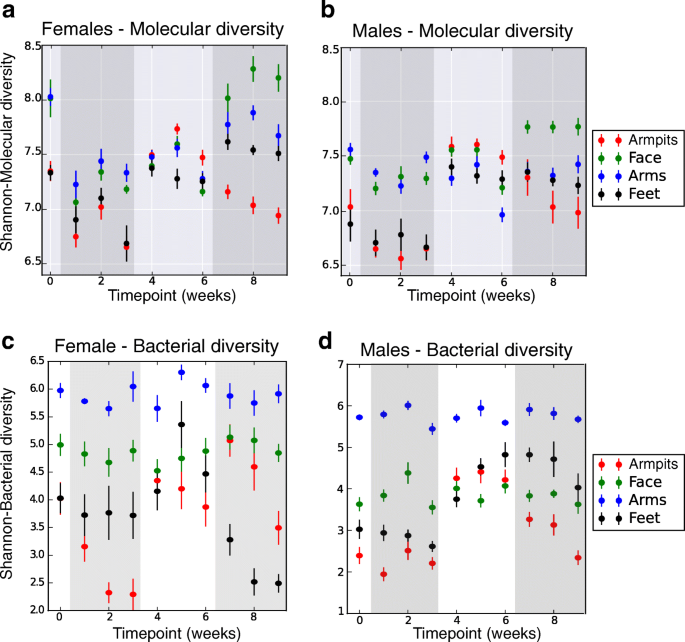
Molecular and bacterial diversity over a 9-week period, comparing samples based on their molecular (UPLC-Q-TOF-MS) or bacterial (16S rRNA amplicon) profiles. Molecular and bacterial diversity using the Shannon index was calculated from samples collected from each body part at each timepoint, separately for female ( n = 5) and male ( n = 6) individuals. Error bars represent standard error of the mean calculated at each timepoint, from up to four samples collected from the right and left side of each body part, of females ( n = 5) and males ( n = 6) separately. a , b Molecular alpha diversity measured using the Shannon index from five females (left panel) and six males (right panel), over 9 weeks, from four distinct body parts (armpits, face, arms, feet). c , d Bacterial alpha diversity measured using the Shannon index, from skin samples collected from five female (left panel) and six male individuals (right panel), over 9 weeks, from four distinct body parts (armpits, face, arms, feet). See also Additional file 1 : Figure S2
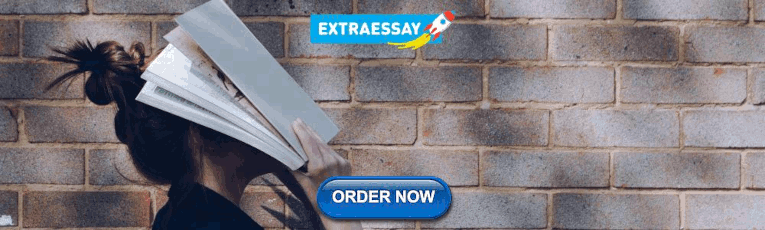
Longitudinal variation of skin metabolomics signatures
To gain insights into temporal metabolomics variation associated with beauty product use, chemical inventories collected over 9 weeks were subjected to multivariate analysis using the widely used Bray–Curtis dissimilarity metric (Fig. 4 a–c, S3A). Throughout the 9-week period, distinct molecular signatures were associated to each specific body site: arm, armpit, face, and foot (Additional file 1 : Figure S3A, Adonis test, p < 0.001, R 2 0.12391). Mass spectrometric signatures displayed distinct individual trends at each specific body site (arm, armpit, face, and foot) over time, supported by their distinct locations in PCoA (principal coordinate analysis) space (Fig. 4 a, b) and based on the Bray–Curtis distances between molecular profiles (Additional file 1 : Figure S3B, WR test, all p values < 0.0001 from T0 through T9). This suggests a high molecular inter-individual variability over time despite similar changes in personal care routines. Significant differences in molecular patterns associated to ceasing (T1–T3) (Fig. 4 b, Additional file 1 : Figure S3C, WR test, T0 vs T1–T3 p < 0.001) and resuming the use of common beauty products (T4–T6) (Additional file 1 : Figure S3C) were observed in the arm, face, and foot (Fig. 4 b), although the armpit exhibited the most pronounced changes (Fig. 4 b, Additional file 1 : Figure S3D, E, random forest highlighting that 100% of samples from each phase were correctly predicted). Therefore, we focused our analysis on this region. Molecular changes were noticeable starting the first week (T1) of discontinuing beauty product use. As shown for armpits in Fig. 4 c, these changes at the chemical level are specific to each individual, possibly due to the extremely personalized lifestyles before the study and match their original use of deodorant. Based on the initial use of underarm products (T0) (Additional file 2 : Table S1), two groups of participants can be distinguished: a group of five volunteers who used stick deodorant as evidenced by the mass spectrometry data and another group of volunteers where we found few or no traces suggesting they never or infrequently used stick deodorants (Additional file 2 : Table S1). Based on this criterion, the chemical trends shown in Fig. 4 c highlight that individuals who used stick deodorant before the beginning of the study (volunteers 1, 2, 3, 9, and 12) displayed a more pronounced shift in their armpits’ chemistries as soon as they stopped using deodorant (T1–T3), compared to individuals who had low detectable levels of stick deodorant use (volunteers 4, 6, 7, and 10), or “rarely-to-never” (volunteers 5 and 11) use stick deodorants as confirmed by the volunteers (Additional file 1 : Figure S3F, WR test, T0 vs T1–T3 all p values < 0.0001, with greater distance for the group of volunteers 1, 2, 3, 9, and 12, compared to volunteers 4, 5, 6, 7, 10, and 11). The most drastic shift in chemical profiles was observed during the transition period, when all participants applied the common antiperspirant on a daily basis (T4–T6) (Additional file 1 : Figure S3D, E). Finally, the molecular profiles became gradually more similar to those collected before the experiment (T0) as soon as the participants resumed using their personal beauty products (T7–T9) (Additional file 1 : Figure S3C), although traces of skin care products did last through the entire T7–T9 period in people who do not routinely apply these products (Fig. 4 c).
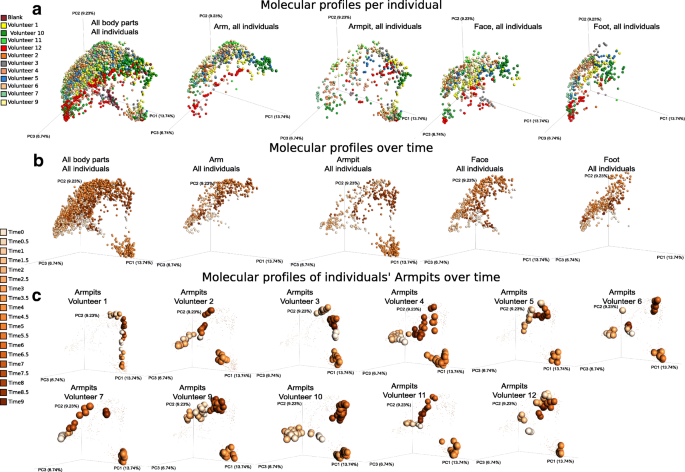
Individualized influence of beauty product application on skin metabolomics profiles over time. a Multivariate statistical analysis (principal coordinate analysis (PCoA)) comparing mass spectrometry data collected over 9 weeks from the skin of 11 individuals, all body parts, combined (first plot from the left) and then displayed separately (arm, armpits, face, feet). Color scale represents volunteer ID. The PCoA was calculated on all samples together, and subsets of the data are shown in this shared space and the other panels. b The molecular profiles collected over 9 weeks from all body parts, combined then separately (arm, armpits, face, feet). c Representative molecular profiles collected over 9 weeks from armpits of 11 individuals (volunteers 1, 2, 3, 4, 5, 6, 7, 9, 10, 11, 12). Color gradient in b and c represents timepoints (time 0 to time 9), ranging from the lightest orange color to the darkest one that represent the earliest (time 0) to the latest (time 9) timepoint, respectively. 0.5 timepoints represent additional timepoints where three selected volunteers were samples (volunteers 4, 9, and 10). PCoA plots were generated using the Bray–Curtis dissimilarity matrix and visualized in Emperor [ 28 ]. See also Additional file 1 : Figure S3
Comparing chemistries detected in armpits at the end timepoints—when no products were used (T3) and during product use (T6)—revealed distinct molecular signatures characteristic of each phase (random forest highlighting that 100% of samples from each group were correctly predicted, see Additional file 1 : Figure S3D, E). Because volunteers used the same antiperspirant during T4–T6, molecular profiles converged during that time despite individual patterns at T3 (Fig. 4 b, c, Additional file 1 : Figure S3D). These distinct chemical patterns reflect the significant impact of beauty products on skin molecular composition. Although these differences may in part be driven by beauty product ingredients detected on the skin (Additional file 1 : Figure S1), we anticipated that additional host- and microbe-derived molecules may also be involved in these molecular changes.
To characterize the chemistries that vary over time, we used molecular networking, a MS visualization approach that evaluates the relationship between MS/MS spectra and compares them to reference MS/MS spectral libraries of known compounds [ 29 , 30 ]. We recently showed that molecular networking can successfully organize large-scale mass spectrometry data collected from the human skin surface [ 18 , 19 ]. Briefly, molecular networking uses the MScluster algorithm [ 31 ] to merge all identical spectra and then compares and aligns all unique pairs of MS/MS spectra based on their similarities where 1.0 indicates a perfect match. Similarities between MS/MS spectra are calculated using a similarity score, and are interpreted as molecular families [ 19 , 24 , 32 , 33 , 34 ]. Here, we used this method to compare and characterize chemistries found in armpits, arms, face, and foot of 11 participants. Based on MS/MS spectral similarities, chemistries highlighted through molecular networking (Additional file 1 : Figure S4A) were associated with each body region with 8% of spectra found exclusively in the arms, 12% in the face, 14% in the armpits, and 2% in the foot, while 18% of the nodes were shared between all four body parts and the rest of spectra were shared between two body sites or more (Additional file 1 : Figure S4B). Greater spectral similarities were highlighted between armpits, face, and arm (12%) followed by the arm and face (9%) (Additional file 1 : Figure S4B).
Molecules were annotated with Global Natural Products Social Molecular Networking (GNPS) libraries [ 29 ], using accurate parent mass and MS/MS fragmentation patterns, according to level 2 or 3 of annotation defined by the 2007 metabolomics standards initiative [ 35 ]. Through annotations, molecular networking revealed that many compounds derived from steroids (Fig. 5 a–d), bile acids (Additional file 1 : Figure S5A-D), and acylcarnitines (Additional file 1 : Figure S5E-F) were exclusively detected in the armpits. Using authentic standards, the identity of some pheromones and bile acids were validated to a level 1 identification with matched retention times (Additional file 1 : Figure S6B, S7A, C, D). Other steroids and bile acids were either annotated using standards with identical MS/MS spectra but slightly different retention times (Additional file 1 : Figure S6A) or annotated with MS/MS spectra match with reference MS/MS library spectra (Additional file 1 : Figure S6C, D, S7B, S6E-G). These compounds were therefore classified as level 3 [ 35 ]. Acylcarnitines were annotated to a family of possible acylcarnitines (we therefore classify as level 3), as the positions of double bonds or cis vs trans configurations are unknown (Additional file 1 : Figure S8A, B).
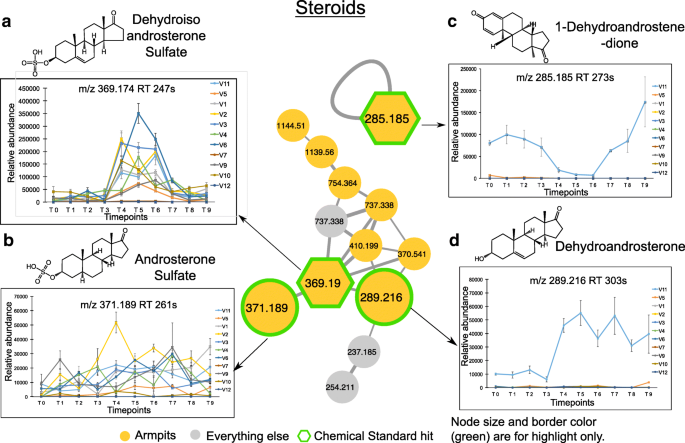
Underarm steroids and their longitudinal abundance. a – d Steroid molecular families in the armpits and their relative abundance over a 9-week period. Molecular networking was applied to characterize chemistries from the skin of 11 healthy individuals. The full network is shown in Additional file 1 : Figure S4A, and networking parameters can be found here http://gnps.ucsd.edu/ProteoSAFe/status.jsp?task=284fc383e4c44c4db48912f01905f9c5 for MS/MS datasets MSV000081582. Each node represents a consensus of a minimum of 3 identical MS/MS spectra. Yellow nodes represent MS/MS spectra detected in armpits samples. Hexagonal shape represents MS/MS spectra match between skin samples and chemical standards. Plots are representative of the relative abundance of each compound over time, calculated separately from LC-MS1 data collected from the armpits of each individual. Steroids detected in armpits are a , dehydroisoandrosterone sulfate ( m/z 369.190, rt 247 s), b androsterone sulfate ( m/z 371.189, rt 261 s), c 1-dehydroandrostenedione ( m/z 285.185, rt 273 s), and d dehydroandrosterone ( m/z 289.216, rt 303 s). Relative abundance over time of each steroid compound is represented. Error bars represent the standard error of the mean calculated at each timepoint from four armpit samples from the right and left side of each individual separately. See also Additional file 1 : Figures S4-S8
Among the steroid compounds, several molecular families were characterized: androsterone (Fig. 5 a, b, d), androstadienedione (Fig. 5 c), androstanedione (Additional file 1 : Figure S6E), androstanolone (Additional file 1 : Figure S6F), and androstenedione (Additional file 1 : Figure S6G). While some steroids were detected in the armpits of several individuals, such as dehydroisoandrosterone sulfate ( m/z 369.19, rt 247 s) (9 individuals) (Fig. 5 a, Additional file 1 : Figure S6A), androsterone sulfate ( m/z 371.189, rt 261 s) (9 individuals) (Fig. 5 b, Additional file 1 : Figure S6C), and 5-alpha-androstane-3,17-dione ( m/z 271.205, rt 249 s) (9 individuals) (Additional file 1 : Figure S6E), other steroids including 1-dehydroandrostenedione ( m/z 285.185, rt 273 s) (Fig. 5 c, Additional file 1 : Figure S6B), dehydroandrosterone ( m/z 289.216, rt 303 s) (Fig. 5 d, Additional file 1 : Figure S6D), and 5-alpha-androstan-17.beta-ol-3-one ( m/z 291.231, rt 318 s) (Additional file 1 : Figure S6F) were only found in the armpits of volunteer 11 and 4-androstene-3,17-dione ( m/z 287.200, rt 293 s) in the armpits of volunteer 11 and volunteer 5, both are male that never applied stick deodorants (Additional file 1 : Figure S6G). Each molecular species exhibited a unique pattern over the 9-week period. The abundance of dehydroisoandrosterone sulfate (Fig. 5 a, WR test, p < 0.01 for 7 individuals) and dehydroandrosterone (Fig. 5 a, WR test, p = 0.00025) significantly increased during the use of antiperspirant (T4–T6), while androsterone sulfate (Fig. 5 b) and 5-alpha-androstane-3,17-dione (Additional file 1 : Figure S6E) display little variation over time. Unlike dehydroisoandrosterone sulfate (Fig. 5 a) and dehydroandrosterone (Fig. 5 d), steroids including 1-dehydroandrostenedione (Fig. 5 c, WR test, p = 0.00024) and 4-androstene-3,17-dione (Additional file 1 : Figure S6G, WR test, p = 0.00012) decreased in abundance during the 3 weeks of antiperspirant application (T4–T6) in armpits of male 11, and their abundance increased again when resuming the use of his normal skin care routines (T7–T9). Interestingly, even within the same individual 11, steroids were differently impacted by antiperspirant use as seen for 1-dehydroandrostenedione that decreased in abundance during T4–T6 (Fig. 5 c, WR test, p = 0.00024), while dehydroandrosterone increased in abundance (Fig. 5 d, WR test, p = 0.00025), and this increase was maintained during the last 3 weeks of the study (T7–T9).
In addition to steroids, many bile acids (Additional file 1 : Figure S5A-D) and acylcarnitines (Additional file 1 : Figure S5E-F) were detected on the skin of several individuals through the 9-week period. Unlike taurocholic acid found only on the face (Additional file 1 : Figures S5A, S7A) and tauroursodeoxycholic acid detected in both armpits and arm samples (Additional file 1 : Figures S5B, S7B), other primary bile acids such as glycocholic (Additional file 1 : Figures S5C, S7C) and chenodeoxyglycocholic acid (Additional file 1 : Figures S5D, S7D) were exclusively detected in the armpits. Similarly, acylcarnitines were also found either exclusively in the armpits (hexadecanoyl carnitines) (Additional file 1 : Figures S5E, S8A) or in the armpits and face (tetradecenoyl carnitine) (Additional file 1 : Figures S5F, S8B) and, just like the bile acids, they were also stably detected during the whole 9-week period.
Bacterial communities and their variation over time
Having demonstrated the impact of beauty products on the chemical makeup of the skin, we next tested the extent to which skin microbes are affected by personal care products. We assessed temporal variation of bacterial communities detected on the skin of healthy individuals by evaluating dissimilarities of bacterial collections over time using unweighted UniFrac distance [ 36 ] and community variation at each body site in association to beauty product use [ 3 , 15 , 37 ]. Unweighted metrics are used for beta diversity calculations because we are primarily concerned with changes in community membership rather than relative abundance. The reason for this is that skin microbiomes can fluctuate dramatically in relative abundance on shorter timescales than that assessed here. Longitudinal variations were revealed for the armpits (Fig. 6 a) and feet microbiome by their overall trend in the PCoA plots (Fig. 6 b), while the arm (Fig. 6 c) and face (Fig. 6 d) displayed relatively stable bacterial profiles over time. As shown in Fig. 6 a–d, although the microbiome was site-specific, it varied more between individuals and this inter-individual variability was maintained over time despite same changes in personal care routine (WR test, all p values at all timepoints < 0.05, T5 p = 0.07), in agreement with previous findings that individual differences in the microbiome are large and stable over time [ 3 , 4 , 10 , 37 ]. However, we show that shifts in the microbiome can be induced by changing hygiene routine and therefore skin chemistry. Changes associated with using beauty products (T4–T6) were more pronounced for the armpits (Fig. 6 a, WR test, p = 1.61e−52) and feet (Fig. 6 b, WR test, p = 6.15e−09), while little variations were observed for the face (Fig. 6 d, WR test, p = 1.402.e−83) and none for the arms (Fig. 6 c, WR test, p = 0.296).
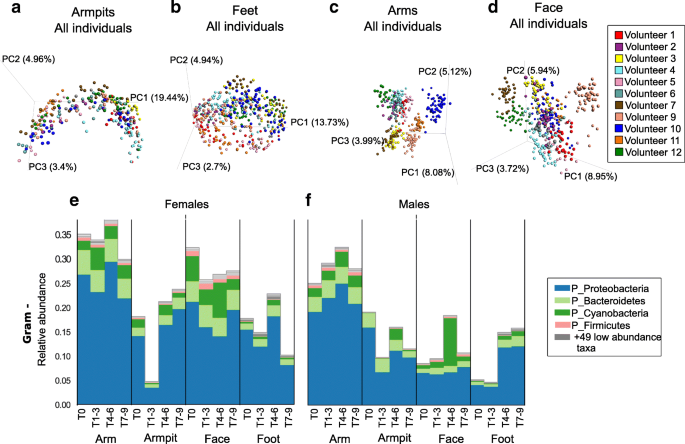
Longitudinal variation of skin bacterial communities in association with beauty product use. a - d Bacterial profiles collected from skin samples of 11 individuals, over 9 weeks, from four distinct body parts a) armpits, b) feet, c) arms and d) face, using multivariate statistical analysis (Principal Coordinates Analysis PCoA) and unweighted Unifrac metric. Each color represents bacterial samples collected from an individual. PCoA were calculated separately for each body part. e , f Representative Gram-negative (Gram -) bacteria collected from arms, armpits, face and feet of e) female and f) male participants. See also Additional file 1 : Figure S9A, B showing Gram-negative bacterial communities represented at the genus level
A significant increase in abundance of Gram-negative bacteria including the phyla Proteobacteria and Bacteroidetes was noticeable for the armpits and feet of both females (Fig. 6 e; Mann–Whitney U , p = 8.458e−07) and males (Fig. 6 f; Mann–Whitney U , p = 0.0004) during the use of antiperspirant (T4–T6), while their abundance remained stable for the arms and face during that time (Fig. 6 e, f; female arm p = 0.231; female face p value = 0.475; male arm p = 0.523;male face p = 6.848751e−07). These Gram-negative bacteria include Acinetobacter and Paracoccus genera that increased in abundance in both armpits and feet of females (Additional file 1 : Figure S9A), while a decrease in abundance of Enhydrobacter was observed in the armpits of males (Additional file 1 : Figure S9B). Cyanobacteria, potentially originating from plant material (Additional file 1 : Figure S9C) also increased during beauty product use (T4–T6) especially in males, in the armpits and face of females (Fig. 6 e) and males (Fig. 6 f). Interestingly, although chloroplast sequences (which group phylogenetically within the cyanobacteria [ 38 ]) were only found in the facial cream (Additional file 1 : Figure S9D), they were detected in other locations as well (Fig. 6 e, f. S9E, F), highlighting that the application of a product in one region will likely affect other regions of the body. For example, when showering, a face lotion will drip down along the body and may be detected on the feet. Indeed, not only did the plant material from the cream reveal this but also the shampoo used for the study for which molecular signatures were readily detected on the feet as well (Additional file 1 : Figure S10A). Minimal average changes were observed for Gram-positive organisms (Additional file 1 : Figure S10B, C), although in some individuals the variation was greater than others (Additional file 1 : Figure S10D, E) as discussed for specific Gram-positive taxa below.
At T0, the armpit’s microflora was dominated by Staphylococcus (26.24%, 25.11% of sequencing reads for females and 27.36% for males) and Corynebacterium genera (26.06%, 17.89% for females and 34.22% for males) (Fig. 7 a—first plot from left and Additional file 1 : Figure S10D, E). They are generally known as the dominant armpit microbiota and make up to 80% of the armpit microbiome [ 39 , 40 ]. When no deodorants were used (T1–T3), an overall increase in relative abundance of Staphylococcus (37.71%, 46.78% for females and 30.47% for males) and Corynebacterium (31.88%, 16.50% for females and 44.15% for males) genera was noticeable (WR test, p < 3.071e−05) (Fig. 7 a—first plot from left), while the genera Anaerococcus and Peptoniphilus decreased in relative abundance (WR test, p < 0.03644) (Fig. 7 a—first plot from left and Additional file 1 : Figure S10D, E). When volunteers started using antiperspirants (T4–T6), the relative abundance of Staphylococcus (37.71%, 46.78% females and 30.47% males, to 21.71%, 25.02% females and 19.25% males) and Corynebacterium (31.88%, 16.50% females and 44.15% males, to 15.83%, 10.76% females and 19.60% males) decreased (WR test, p < 3.071e−05) (Fig. 7 a, Additional file 1 : Figure S10D, E) and at the same time, the overall alpha diversity increased significantly (WR test, p = 3.47e−11) (Fig. 3 c, d). The microbiota Anaerococcus (WR test, p = 0.0006018) , Peptoniphilus (WR test, p = 0.008639), and Micrococcus (WR test, p = 0.0377) increased significantly in relative abundance, together with a lot of additional low-abundant species that lead to an increase in Shannon alpha diversity (Fig. 3 c, d). When participants went back to normal personal care products (T7–T9), the underarm microbiome resembled the original underarm community of T0 (WR test, p = 0.7274) (Fig. 7 a). Because armpit bacterial communities are person-specific (inter-individual variability: WR test, all p values at all timepoints < 0.05, besides T5 p n.s), variation in bacterial abundance upon antiperspirant use (T4–T6) differ between individuals and during the whole 9-week period (Fig. 7a —taxonomic plots per individual). For example, the underarm microbiome of male 5 exhibited a unique pattern, where Corynebacterium abundance decreased drastically during the use of antiperspirant (82.74 to 11.71%, WR test, p = 3.518e−05) while in the armpits of female 9 a huge decrease in Staphylococcus abundance was observed (Fig. 7 a) (65.19 to 14.85%, WR test, p = 0.000113). Unlike other participants, during T0–T3, the armpits of individual 11 were uniquely characterized by the dominance of a sequence that matched most closely to the Enhydrobacter genera . The transition to antiperspirant use (T4–T6) induces the absence of Enhydrobacter (30.77 to 0.48%, WR test, p = 0.01528) along with an increase of Corynebacterium abundance (26.87 to 49.74%, WR test, p = 0.1123) (Fig. 7 a—male 11).
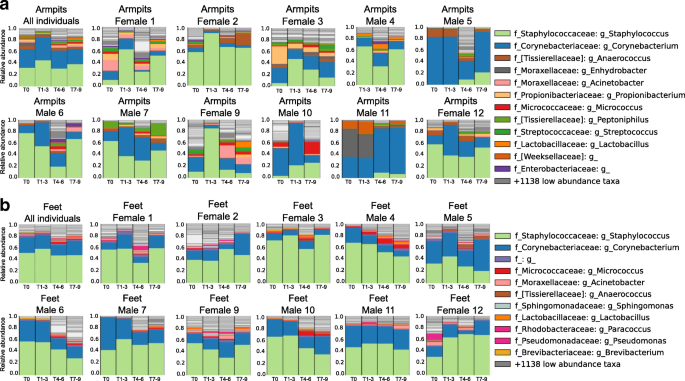
Person-to-person bacterial variabilities over time in the armpits and feet. a Armpit microbiome changes when stopping personal care product use, then resuming. Armpit bacterial composition of the 11 volunteers combined, then separately, (female 1, female 2, female 3, male 4, male 5, male 6, male 7, female 9, male 10, male 11, female 12) according to the four periods within the experiment. b Feet bacterial variation over time of the 12 volunteers combined, then separately (female 1, female 2, female 3, male 4, male 5, male 6, male 7, female 9, male 10, male 11, female 12) according to the four periods within the experiment. See also Additional file 1 : Figure S9-S13
In addition to the armpits, a decline in abundance of Staphylococcus and Corynebacterium was perceived during the use of the foot powder (46.93% and 17.36%, respectively) compared to when no beauty product was used (58.35% and 22.99%, respectively) (WR test, p = 9.653e−06 and p = 0.02032, respectively), while the abundance of low-abundant foot bacteria significantly increased such as Micrococcus (WR test, p = 1.552e−08), Anaerococcus (WR test, p = 3.522e−13), Streptococcus (WR test, p = 1.463e−06), Brevibacterium (WR test, p = 6.561e−05), Moraxellaceae (WR test, p = 0.0006719), and Acinetobacter (WR test, p = 0.001487), leading to a greater bacterial diversity compared to other phases of the study (Fig. 7 b first plot from left, Additional file 1 : Figure S10D, E, Fig. 3 c, d).
We further evaluated the relationship between the two omics datasets by superimposing the principal coordinates calculated from metabolome and microbiome data (Procrustes analysis) (Additional file 1 : Figure S11) [ 34 , 41 , 42 ]. Metabolomics data were more correlated with patterns observed in microbiome data in individual 3 (Additional file 1 : Figure S11C, Mantel test, r = 0.23, p < 0.001), individual 5 (Additional file 1 : Figure S11E, r = 0.42, p < 0.001), individual 9 (Additional file 1 : Figure S11H, r = 0.24, p < 0.001), individual 10 (Additional file 1 : Figure S11I, r = 0.38, p < 0.001), and individual 11 (Additional file 1 : Figure S11J, r = 0.35, p < 0.001) when compared to other individuals 1, 2, 4, 6, 7, and 12 (Additional file 1 : Figure S11A, B, D, F, G, K, respectively) (Mantel test, all r < 0.2, all p values < 0.002, for volunteer 2 p n.s). Furthermore, these correlations were individually affected by ceasing (T1–T3) or resuming the use of beauty products (T4–T6 and T7–T9) (Additional file 1 : Figure S11A-K).
Overall, metabolomics–microbiome correlations were consistent over time for the arms, face, and feet although alterations were observed in the arms of volunteers 7 (Additional file 1 : Figure S11G) and 10 (Additional file 1 : Figure S11I) and the face of volunteer 7 (Additional file 1 : Figure S11G) during product use (T4–T6). Molecular–bacterial correlations were mostly affected in the armpits during antiperspirant use (T4–T6), as seen for volunteers male 7 (Additional file 1 : Figure S11G) and 11 (Additional file 1 : Figure S11J) and females 2 (Additional file 1 : Figure S11B), 9 (Additional file 1 : Figure S11H), and 12 (Additional file 1 : Figure S11K). This perturbation either persisted during the last 3 weeks (Additional file 1 : Figure S11D, E, H, I, K) when individuals went back to their normal routine (T7–T9) or resembled the initial molecular–microbial correlation observed in T0 (Additional file 1 : Figure S11C, G, J). These alterations in molecular–bacterial correlation are driven by metabolomics changes during antiperspirant use as revealed by metabolomics shifts on the PCoA space (Additional file 1 : Figure S11), partially due to the deodorant’s chemicals (Additional file 1 : Figure S1J, K) but also to changes observed in steroid levels in the armpits (Fig. 5A, C, D , Additional file 1 : Figure S6G), suggesting metabolome-dependant changes of the skin microbiome. In agreement with previous findings that showed efficient biotransformation of steroids by Corynebacterium [ 43 , 44 ], our correlation analysis associates specific steroids that were affected by antiperspirant use in the armpits of volunteer 11 (Fig. 5 c, d, Additional file 1 : Figure S6G) with microbes that may produce or process them: 1-dehydroandrostenedione, androstenedione, and dehydrosterone with Corynebacterium ( r = − 0.674, p = 6e−05; r = 0.671, p = 7e−05; r = 0.834, p < 1e−05, respectively) (Additional file 1 : Figure S12A, B, C, respectively) and Enhydrobacter ( r = 0.683, p = 4e−05; r = 0.581, p = 0.00095; r = 0.755, p < 1e−05 respectively) (Additional file 1 : Figure S12D, E, F, respectively).
Despite the widespread use of skin care and hygiene products, their impact on the molecular and microbial composition of the skin is poorly studied. We established a workflow that examines individuals to systematically study the impact of such lifestyle characteristics on the skin by taking a broad look at temporal molecular and bacterial inventories and linking them to personal skin care product use. Our study reveals that when the hygiene routine is modified, the skin metabolome and microbiome can be altered, but that this alteration depends on product use and location on the body. We also show that like gut microbiome responses to dietary changes [ 20 , 21 ], the responses are individual-specific.
We recently reported that traces of our lifestyle molecules can be detected on the skin days and months after the original application [ 18 , 19 ]. Here, we show that many of the molecules associated with our personal skin and hygiene products had a half-life of 0.5 to 1.9 weeks even though the volunteers regularly showered, swam, or spent time in the ocean. Thus, a single application of some of these products has the potential to alter the microbiome and skin chemistry for extensive periods of time. Our data suggests that although host genetics and diet may play a role, a significant part of the resilience of the microbiome that has been reported [ 10 , 45 ] is due to the resilience of the skin chemistry associated with personal skin and hygiene routines, or perhaps even continuous re-exposure to chemicals from our personal care routines that are found on mattresses, furniture, and other personal objects [ 19 , 27 , 46 ] that are in constant contact. Consistent with this observation is that individuals in tribal regions and remote villages that are infrequently exposed to the types of products used in this study have very different skin microbial communities [ 47 , 48 ] and that the individuals in this study who rarely apply personal care products had a different starting metabolome. We observed that both the microbiome and skin chemistry of these individuals were most significantly affected by these products. This effect by the use of products at T4–T6 on the volunteers that infrequently used them lasted to the end phase of the study even though they went back to infrequent use of personal care products. What was notable and opposite to what the authors originally hypothesized is that the use of the foot powder and antiperspirant increased the diversity of microbes and that some of this diversity continued in the T7–T9 phase when people went back to their normal skin and hygiene routines. It is likely that this is due to the alteration in the nutrient availability such as fatty acids and moisture requirements, or alteration of microbes that control the colonization via secreted small molecules, including antibiotics made by microbes commonly found on the skin [ 49 , 50 ].
We detected specific molecules on the skin that originated from personal care products or from the host. One ingredient that lasts on the skin is propylene glycol, which is commonly used in deodorants and antiperspirants and added in relatively large amounts as a humectant to create a soft and sleek consistency [ 51 ]. As shown, daily use of personal care products is leading to high levels of exposure to these polymers. Such polymers cause contact dermatitis in a subset of the population [ 51 , 52 ]. Our data reveal a lasting accumulation of these compounds on the skin, suggesting that it may be possible to reduce their dose in deodorants or frequency of application and consequently decrease the degree of exposure to such compounds. Formulation design of personal care products may be influenced by performing detailed outcome studies. In addition, longer term impact studies are needed, perhaps in multiple year follow-up studies, to assess if the changes we observed are permanent or if they will recover to the original state.
Some of the host- and microbiome-modified molecules were also detected consistently, such as acylcarnitines, bile acids, and certain steroids. This means that a portion of the molecular composition of a person’s skin is not influenced by the beauty products applied to the skin, perhaps reflecting the level of exercise for acylcarnitines [ 53 , 54 ] or the liver (dominant location where they are made) or gallbladder (where they are stored) function for bile acids. The bile acid levels are not related to sex and do not change in amount during the course of this study. While bile acids are typically associated with the human gut microbiome [ 34 , 55 , 56 , 57 , 58 ], it is unclear what their role is on the skin and how they get there. One hypothesis is that they are present in the sweat that is excreted through the skin, as this is the case for several food-derived molecules such as caffeine or drugs and medications that have been previously reported on the human skin [ 19 ] or that microbes synthesize them de novo [ 55 ]. The only reports we could find on bile acids being associated with the skin describe cholestasis and pruritus diseases. Cholestasis and pruritus in hepatobiliary disease have symptoms of skin bile acid accumulation that are thought to be responsible for severe skin itching [ 59 , 60 ]. However, since bile acids were found in over 50% of the healthy volunteers, their detection on the skin is likely a common phenotype among the general population and not only reflective of disease, consistent with recent reports challenging these molecules as biomarkers of disease [ 59 ]. Other molecules that were detected consistently came from personal care products.
Aside from molecules that are person-specific and those that do not vary, there are others that can be modified via personal care routines. Most striking is how the personal care routines influenced changes in hormones and pheromones in a personalized manner. This suggests that there may be personalized recipes that make it possible to make someone more or less attractive to others via adjustments of hormonal and pheromonal levels through alterations in skin care.
Here, we describe the utilization of an approach that combines metabolomics and microbiome analysis to assess the effect of modifying personal care regime on skin chemistry and microbes. The key findings are as follows: (1) Compounds from beauty products last on the skin for weeks after their first use despite daily showering. (2) Beauty products alter molecular and bacterial diversity as well as the dynamic and structure of molecules and bacteria on the skin. (3) Molecular and bacterial temporal variability is product-, site-, and person-specific, and changes are observed starting the first week of beauty product use. This study provides a framework for future investigations to understand how lifestyle characteristics such as diet, outdoor activities, exercise, and medications shape the molecular and microbial composition of the skin. These factors have been studied far more in their impact on the gut microbiome and chemistry than in the skin. Revealing how such factors can affect skin microbes and their associated metabolites may be essential to define long-term skin health by restoring the appropriate microbes particularly in the context of skin aging [ 61 ] and skin diseases [ 49 ] as has shown to be necessary for amphibian health [ 62 , 63 ], or perhaps even create a precision skin care approach that utilizes the proper care ingredients based on the microbial and chemical signatures that could act as key players in host defense [ 49 , 64 , 65 ].
Subject recruitment and sample collection
Twelve individuals between 25 and 40 years old were recruited to participate in this study, six females and six males. Female volunteer 8 dropped out of the study as she developed a skin irritation during the T1–T3 phase. All volunteers signed a written informed consent in accordance with the sampling procedure approved by the UCSD Institutional Review Board (Approval Number 161730). Volunteers were required to follow specific instructions during 9 weeks. They were asked to bring in samples of their personal care products they used prior to T0 so they could be sampled as well. Following the initial timepoint time 0 and during the first 3 weeks (week 1–week 3), volunteers were asked not to use any beauty products (Fig. 1 b). During the next 3 weeks (week 4–week 6), four selected commercial beauty products provided to all volunteers were applied once a day at specific body part (deodorant for the armpits, soothing foot powder between the toes, sunscreen for the face, and moisturizer for front forearms) (Fig. 1 b, Additional file 3 : Table S2 Ingredient list of beauty products). During the first 6 weeks, volunteers were asked to shower with a head to toe shampoo. During the last 3 weeks (week 7–week 9), all volunteers went back to their normal routine and used the personal care products used before the beginning of the study (Fig. 1 b). Volunteers were asked not to shower the day before sampling. Samples were collected by the same three researchers to ensure consistency in sampling and the area sampled. Researchers examined every subject together and collected metabolomics and microbiome samples from each location together. Samples were collected once a week (from day 0 to day 68—10 timepoints total) for volunteers 1, 2, 3, 4, 5, 6, 7, 9, 10, 11, and 12, and on day 0 and day 6 for volunteer 8. For individuals 4, 9, and 10, samples were collected twice a week. Samples collected for 11 volunteers during 10 timepoints: 11 volunteers × 10 timepoints × 4 samples × 4 body sites = 1760. Samples collected from 3 selected volunteers during 9 additional timepoints: 3 volunteers × 9 timepoints × 4 samples × 4 body sites = 432. All samples were collected following the same protocol described in [ 18 ]. Briefly, samples were collected over an area of 2 × 2 cm, using pre-moistened swabs in 50:50 ethanol/water solution for metabolomics analysis or in Tris-EDTA buffer for 16S rRNA sequencing. Four samples were collected from each body part right and left side. The locations sampled were the face—upper cheek bone and lower jaw, armpit—upper and lower area, arm—front of the elbow (antecubitis) and forearm (antebrachium), and feet—in between the first and second toe and third and fourth toe. Including personal care product references, a total of 2275 samples were collected over 9 weeks and were submitted to both metabolomics and microbial inventories.
Metabolite extraction and UPLC-Q-TOF mass spectrometry analysis
Skin swabs were extracted and analyzed using a previously validated workflow described in [ 18 , 19 ]. All samples were extracted in 200 μl of 50:50 ethanol/water solution for 2 h on ice then overnight at − 20 °C. Swab sample extractions were dried down in a centrifugal evaporator then resuspended by vortexing and sonication in a 100 μl 50:50 ethanol/water solution containing two internal standards (fluconazole 1 μM and amitriptyline 1 μM). The ethanol/water extracts were then analyzed using a previously validated UPLC-MS/MS method [ 18 , 19 ]. We used a ThermoScientific UltiMate 3000 UPLC system for liquid chromatography and a Maxis Q-TOF (Quadrupole-Time-of-Flight) mass spectrometer (Bruker Daltonics), controlled by the Otof Control and Hystar software packages (Bruker Daltonics) and equipped with ESI source. UPLC conditions of analysis are 1.7 μm C18 (50 × 2.1 mm) UHPLC Column (Phenomenex), column temperature 40 °C, flow rate 0.5 ml/min, mobile phase A 98% water/2% acetonitrile/0.1% formic acid ( v / v ), mobile phase B 98% acetonitrile/2% water/0.1% formic acid ( v / v ). A linear gradient was used for the chromatographic separation: 0–2 min 0–20% B, 2–8 min 20–99% B, 8–9 min 99–99% B, 9–10 min 0% B. Full-scan MS spectra ( m/z 80–2000) were acquired in a data-dependant positive ion mode. Instrument parameters were set as follows: nebulizer gas (nitrogen) pressure 2 Bar, capillary voltage 4500 V, ion source temperature 180 °C, dry gas flow 9 l/min, and spectra rate acquisition 10 spectra/s. MS/MS fragmentation of 10 most intense selected ions per spectrum was performed using ramped collision induced dissociation energy, ranged from 10 to 50 eV to get diverse fragmentation patterns. MS/MS active exclusion was set after 4 spectra and released after 30 s.
Mass spectrometry data collected from the skin of 12 individuals can be found here MSV000081582.
LC-MS data processing
LC-MS raw data files were converted to mzXML format using Compass Data analysis software (Bruker Daltonics). MS1 features were selected for all LC-MS datasets collected from the skin of 12 individuals and blank samples (total 2275) using the open-source software MZmine [ 66 ]—see Additional file 4 : Table S3 for parameters. Subsequent blank filtering, total ion current, and internal standard normalization were performed (Additional file 5 : Table S4) for representation of relative abundance of molecular features (Fig. 2 , Additional file 1 : Figure S1), principal coordinate analysis (PCoA) (Fig. 4 ). For steroid compounds in Fig. 5 a–d, bile acids (Additional file 1 : Figure S5A-D), and acylcarnitines (Additional file 1 : Figure S5E, F) compounds, crop filtering feature available in MZmine [ 66 ] was used to identify each feature separately in all LC-MS data collected from the skin of 12 individuals (see Additional file 4 : Table S3 for crop filtering parameters and feature finding in Additional file 6 : Table S5).
Heatmap in Fig. 2 was constructed from the bucket table generated from LC-MS1 features (Additional file 7 : Table S6) and associated metadata (Additional file 8 : Table S7) using the Calour command line available here: https://github.com/biocore/calour . Calour parameters were as follows: normalized read per sample 5000 and cluster feature minimum reads 50. Procrustes and Pearson correlation analyses in Additional file 1 : Figures S10 and S11 were performed using the feature table in Additional file 9 : Table S8, normalized using the probabilistic quotient normalization method [ 67 ].
16S rRNA amplicon sequencing
16S rRNA sequencing was performed following the Earth Microbiome Project protocols [ 68 , 69 ], as described before [ 18 ]. Briefly, DNA was extracted using MoBio PowerMag Soil DNA Isolation Kit and the V4 region of the 16S rRNA gene was amplified using barcoded primers [ 70 ]. PCR was performed in triplicate for each sample, and V4 paired-end sequencing [ 70 ] was performed using Illumina HiSeq (La Jolla, CA). Raw sequence reads were demultiplexed and quality controlled using the defaults, as provided by QIIME 1.9.1 [ 71 ]. The primary OTU table was generated using Qiita ( https://qiita.ucsd.edu/ ), using UCLUST ( https://academic.oup.com/bioinformatics/article/26/19/2460/230188 ) closed-reference OTU picking method against GreenGenes 13.5 database [ 72 ]. Sequences can be found in EBI under accession number EBI: ERP104625 or in Qiita ( qiita.ucsd.edu ) under Study ID 10370. Resulting OTU tables were then rarefied to 10,000 sequences/sample for downstream analyses (Additional file 10 Table S9). See Additional file 11 : Table S10 for read count per sample and Additional file 1 : Figure S13 representing the samples that fall out with rarefaction at 10,000 threshold. The dataset includes 35 blank swab controls and 699 empty controls. The blank samples can be accessed through Qiita ( qiita.ucsd.edu ) as study ID 10370 and in EBI with accession number EBI: ERP104625. Blank samples can be found under the metadata category “sample_type” with the name “empty control” and “Swabblank.” These samples fell below the rarefaction threshold at 10,000 (Additional file 11 : Table S10).
To rule out the possibility that personal care products themselves contained the microbes that induced the changes in the armpit and foot microbiomes that were observed in this study (Fig. 7 ), we subjected the common personal care products that were used in this study during T4–T6 also to 16S rRNA sequencing. The data revealed that within the limit of detectability of the current experiment, few 16S signatures were detected. One notable exception was the most dominant plant-originated bacteria chloroplast detected in the sunscreen lotion applied on the face (Additional file 1 : Figure S9D), that was also detected on the face of individuals and at a lower level on their arms, sites where stable microbial communities were observed over time (Additional file 1 : Figure S9E, F). This finding is in agreement with our previous data from the 3D cartographical skin maps that revealed the presence of co-localized chloroplast and lotion molecules [ 18 ]. Other low-abundant microbial signatures found in the sunscreen lotion include additional plant-associated bacteria: mitochondria [ 73 ], Bacillaceae [ 74 , 75 ], Planococcaceae [ 76 ], and Ruminococcaceae family [ 77 ], but all these bacteria are not responsible for microbial changes associated to beauty product use, as they were poorly detected in the armpits and feet (Fig. 7 ).
To assess the origin of Cyanobacteria detected in skin samples, each Greengenes [ 72 ] 13_8 97% OTU table (per lane; obtained from Qiita [ 78 ] study 10,370) was filtered to only features with a p__Cyanobacteria phylum. The OTU maps for these tables—which relate each raw sequence to an OTU ID—were then filtered to only those observed p__Cyanobacteria OTU IDs. The filtered OTU map was used to extract the raw sequences into a single file. Separately, the unaligned Greengenes 13_8 99% representative sequences were filtered into two sets, first the set of representatives associated with c__Chloroplast (our interest database), and second the set of sequences associated with p__Cyanobacteria without the c__Chloroplast sequences (our background database). Platypus Conquistador [ 79 ] was then used to determine what reads were observed exclusively in the interest database and not in the background database. Of the 4,926,465 raw sequences associated with a p__Cyanobacteria classification (out of 318,686,615 total sequences), at the 95% sequence identity level with 100% alignment, 4,860,258 sequences exclusively recruit to full-length chloroplast 16S by BLAST [ 80 ] with the bulk recruiting to streptophytes (with Chlorophyta and Stramenopiles to a lesser extent). These sequences do not recruit non-chloroplast Cyanobacteria full length 16S.
Half-life calculation for metabolomics data
In order to estimate the biological half-life of molecules detected in the skin, the first four timepoints of the study (T0, T1, T2, T3) were considered for the calculation to allow the monitoring of personal beauty products used at T0. The IUPAC’s definition of biological half-life as the time required to a substance in a biological system to be reduced to half of its value, assuming an approximately exponential removal [ 81 ] was used. The exponential removal can be described as C ( t ) = C 0 e − tλ where t represents the time in weeks, C 0 represents the initial concentration of the molecule, C ( t ) represents the concentration of the molecule at time t , and λ is the rate of removal [ http://onlinelibrary.wiley.com/doi/10.1002/9780470140451.ch2/summary ]. The parameter λ was estimated by a mixed linear effects model in order to account for the paired sample structure. The regression model tests the null hypothesis that λ is equal to zero and only the significant ( p value < 0.05) parameters were considered.
Principal coordinate analysis
We performed principal coordinate analysis (PCoA) on both metabolomics and microbiome data. For metabolomics, we used MS1 features (Additional file 5 : Table S4) and calculated Bray–Curtis dissimilarity metric using ClusterApp ( https://github.com/mwang87/q2_metabolomics ).
For microbiome data, we used rarefied OTU table (Additional file 10 : Table S9) and used unweighted UniFrac metric [ 36 ] to calculate beta diversity distance matrix using QIIME2 (https://qiime2.org). Results from both data sources were visualized using Emperor ( https://biocore.github.io/emperor/ ) [ 28 ].
Molecular networking
Molecular networking was generated from LC-MS/MS data collected from skin samples of 11 individuals MSV000081582, using the Global Natural Products Social Molecular Networking platform (GNPS) [ 29 ]. Molecular network parameters for MS/MS data collected from all body parts of 11 individuals during T0–T9 MSV000081582 are accessible here http://gnps.ucsd.edu/ProteoSAFe/status.jsp?task=284fc383e4c44c4db48912f01905f9c5 . Molecular network parameters for MS/MS data collected from armpits T0–T3 MSV000081582 and deodorant used by individual 1 and 3 MSV000081580 can be found here http://gnps.ucsd.edu/ProteoSAFe/status.jsp?task=f5325c3b278a46b29e8860ec57915ad and here http://gnps.ucsd.edu/ProteoSAFe/status.jsp?task=aaa1af68099d4c1a87e9a09f398fe253 , respectively. Molecular networks were exported and visualized in Cytoscape 3.4.0. [ 82 ]. Molecular networking parameters were set as follows: parent mass tolerance 1 Da, MS/MS fragment ion tolerance 0.5 Da, and cosine threshold 0.65 or greater, and only MS/MS spectral pairs with at least 4 matched fragment ions were included. Each MS/MS spectrum was only allowed to connect to its top 10 scoring matches, resulting in a maximum of 10 connections per node. The maximum size of connected components allowed in the network was 600, and the minimum number of spectra required in a cluster was 3. Venn diagrams were generated from Cytoscape data http://gnps.ucsd.edu/ProteoSAFe/status.jsp?task=284fc383e4c44c4db48912f01905f9c5 using Cytoscape [ 82 ] Venn diagram app available here http://apps.cytoscape.org/apps/all .
Shannon molecular and bacterial diversity
The diversity analysis was performed separately for 16S rRNA data and LC-MS data. For each sample in each feature table (LC-MS data and microbiome data), we calculated the value of the Shannon diversity index. For LC-MS data, we used the full MZmine feature table (Additional file 5 : Table S4). For microbiome data, we used the closed-reference BIOM table rarefied to 10,000 sequences/sample. For diversity changes between timepoints, we aggregated Shannon diversity values across groups of individuals (all, females, males) and calculated mean values and standard errors. All successfully processed samples (detected features in LC-MS or successful sequencing with 10,000 or more sequences/sample) were considered.
Beauty products and chemical standards
Samples (10 mg) from personal care products used during T0 and T7–T9 MSV000081580 (Additional file 2 : Table S1) and common beauty products used during T4–T6 MSV000081581 (Additional file 3 : Table S2) were extracted in 1 ml 50:50 ethanol/water. Sample extractions were subjected to the same UPLC-Q-TOF MS method used to analyze skin samples and described above in the section “ Metabolite extraction and UPLC-Q-TOF mass spectrometry analysis .” Authentic chemical standards MSV000081583 including 1-dehydroandrostenedion (5 μM), chenodeoxyglycocholic acid (5 μM), dehydroisoandrosterone sulfate (100 μM), glycocholic acid (5 μM), and taurocholic acid (5 μM) were analyzed using the same mass spectrometry workflow used to run skin and beauty product samples.
Monitoring beauty product ingredients in skin samples
In order to monitor beauty product ingredients used during T4–T6, we selected only molecular features present in each beauty product sample (antiperspirant, facial lotion, body moisturizer, soothing powder) and then filtered the aligned MZmine feature table (Additional file 5 : Table S4) for the specific feature in specific body part samples. After feature filtering, we selected all features that had a higher average intensity on beauty product phase (T4–T6) compared to non-beauty product phase (T1–T3). The selected features were annotated using GNPS dereplication output http://gnps.ucsd.edu/ProteoSAFe/status.jsp?task=69319caf219642a5a6748a3aba8914df , plotted using R package ggplot2 ( https://cran.r-project.org/web/packages/ggplot2/index.html ) and visually inspected for meaningful patterns.
Random forest analysis
Random forest analysis was performed in MetaboAnalyst 3.0 online platform http://www.metaboanalyst.ca/faces/home.xhtml . Using LC-MS1 features found in armpit samples collected on T3 and T6. Random forest parameters were set as follows: top 1000 most abundant features, number of predictors to try for each node 7, estimate of error rate (0.0%).
BugBase analysis
To determine the functional potential of microbial communities within our samples, we used BugBase [ 83 ]. Because we do not have direct access to all of the gene information due to the use of 16S rRNA marker gene sequencing, we can only rely on phylogenetic information inferred from OTUs. BugBase takes advantage of this information to predict microbial phenotypes by associating OTUs with gene content using PICRUSt [ 84 ]. Thus, using BugBase, we can predict such phenotypes as Gram staining, or oxidative stress tolerance at each timepoint or each phase. All statistical analyses in BugBase are performed using non-parametric differentiation tests (Mann–Whitney U ).
Taxonomic plots
Rarefied OTU counts were collapsed according to the OTU’s assigned family and genus name per sample, with a single exception for the class of chloroplasts. Relative abundances of each family-genus group are obtained by dividing by overall reads per sample, i.e., 10,000. Samples are grouped by volunteer, body site, and time/phase. Abundances are aggregated by taking the mean overall samples, and resulting abundances are again normalized to add up to 1. Low-abundant taxa are not listed in the legend and plotted in grayscale. Open-source code is available at https://github.com/sjanssen2/ggmap/blob/master/ggmap/snippets.py
Dissimilarity-based analysis
Pairwise dissimilarity matrices were generated for metabolomics and 16S metagenomics quantification tables, described above, using Bray–Curtis dissimilarity through QIIME 1.9.1 [ 71 ]. Those distance matrices were used to perform Procrustes analysis (QIIME 1.9.1), and Mantel test (scikit-bio version 0.5.1) to measure the correlation between the metabolome and microbiome over time. The metabolomics dissimilarities were used to perform the PERMANOVA test to assess the significance of body part grouping. The PCoA and Procrustes plots were visualized in EMPeror. The dissimilarity matrices were also used to perform distance tests, comparing the distances within and between individuals and distances from time 0 to times 1, 2, and 3 using Wilcoxon rank-sum tests (SciPy version 0.19.1) [ 19 ].
Statistical analysis for molecular and microbial data
Statistical analyses were performed in R and Python (R Core Team 2018). Monotonic relationships between two variables were tested using non-parametric Spearman correlation tests. The p values for correlation significance were subsequently corrected using Benjamini and Hochberg false discovery rate control method. The relationship between two groups was tested using non-parametric Wilcoxon rank-sum tests. The relationship between multiple groups was tested using non-parametric Kruskal–Wallis test. The significance level was set to 5%, unless otherwise mentioned, and all tests were performed as two-sided tests.
Oh J, Byrd AL, Deming C, Conlan S, Kong HH, Segre JA. Biogeography and individuality shape function in the human skin metagenome. Nature. 2014;514(7520):59–64.
Article CAS PubMed PubMed Central Google Scholar
Grice EA, Segre JA. The skin microbiome. Nat Rev Microbiol. 2011;9(4):244–53.
Costello EK, Lauber CL, Hamady M, Fierer N, Gordon JI, Knight R. Bacterial community variation in human body habitats across space and time. Science. 2009;326(5960):1694–7.
Grice EA, Kong HH, Conlan S, Deming CB, Davis J, Young AC, et al. Topographical and temporal diversity of the human skin microbiome. Science. 2009;324(5931):1190–2.
Urban J, Fergus DJ, Savage AM, Ehlers M, Menninger HL, Dunn RR, et al. The effect of habitual and experimental antiperspirant and deodorant product use on the armpit microbiome. PeerJ. 2016;4:e1605.
Article PubMed PubMed Central Google Scholar
Callewaert C, Hutapea P, Van de Wiele T, Boon N. Deodorants and antiperspirants affect the axillary bacterial community. Arch Dermatol Res. 2014;306(8):701–10.
Article CAS PubMed Google Scholar
Staudinger T, Pipal A, Redl B. Molecular analysis of the prevalent microbiota of human male and female forehead skin compared to forearm skin and the influence of make-up. J Appl Microbiol. 2011;110(6):1381–9.
Houben E, De Paepe K, Rogiers V. A keratinocyte’s course of life. Skin Pharmacol Physiol. 2007;20(3):122–32.
Hoath SB, Leahy DG. The organization of human epidermis: functional epidermal units and phi proportionality. J Invest Dermatol. 2003;121(6):1440–6.
Oh J, Byrd AL, Park M, Kong HH, Segre JA. Temporal stability of the human skin microbiome. Cell. 2016;165(4):854–66.
Schloissnig S, Arumugam M, Sunagawa S, Mitreva M, Tap J, Zhu A, et al. Genomic variation landscape of the human gut microbiome. Nature. 2013;493(7430):45–50.
Article PubMed Google Scholar
Faith JJ, Guruge JL, Charbonneau M, Subramanian S, Seedorf H, Goodman AL, et al. The long-term stability of the human gut microbiota. Science. 2013;341(6141):1237439.
Hall MW, Singh N, Ng KF, Lam DK, Goldberg MB, Tenenbaum HC, et al. Inter-personal diversity and temporal dynamics of dental, tongue, and salivary microbiota in the healthy oral cavity. NPJ Biofilms Microbiomes. 2017;3:2.
Utter DR, Mark Welch JL, Borisy GG. Individuality, stability, and variability of the plaque microbiome. Front Microbiol. 2016;7:564.
Flores GE, Caporaso JG, Henley JB, Rideout JR, Domogala D, Chase J, et al. Temporal variability is a personalized feature of the human microbiome. Genome Biol. 2014;15(12):531.
The Human Microbiome Project C. Structure, function and diversity of the healthy human microbiome. Nature [Article]. 2012;486:207.
Article Google Scholar
Dorrestein PC, Gallo RL, Knight R. Microbial skin inhabitants: friends forever. Cell. 2016;165(4):771–2.
Bouslimani A, Porto C, Rath CM, Wang M, Guo Y, Gonzalez A, et al. Molecular cartography of the human skin surface in 3D. Proc Natl Acad Sci U S A. 2015;112(17):E2120–9.
Bouslimani A, Melnik AV, Xu Z, Amir A, da Silva RR, Wang M, et al. Lifestyle chemistries from phones for individual profiling. Proc Natl Acad Sci U S A. 2016;113(48):E7645–E54.
David LA, Maurice CF, Carmody RN, Gootenberg DB, Button JE, Wolfe BE, et al. Diet rapidly and reproducibly alters the human gut microbiome. Nature. 2014;505(7484):559–63.
Wu GD, Chen J, Hoffmann C, Bittinger K, Chen YY, Keilbaugh SA, et al. Linking long-term dietary patterns with gut microbial enterotypes. Science. 2011;334(6052):105–8.
Unno M, Cho O, Sugita T. Inhibition of Propionibacterium acnes lipase activity by the antifungal agent ketoconazole. Microbiol Immunol. 2017;61(1):42–4.
Holland C, Mak TN, Zimny-Arndt U, Schmid M, Meyer TF, Jungblut PR, et al. Proteomic identification of secreted proteins of Propionibacterium acnes. BMC Microbiol. 2010;10:230.
Nguyen DD, Wu CH, Moree WJ, Lamsa A, Medema MH, Zhao X, et al. MS/MS networking guided analysis of molecule and gene cluster families. Proc Natl Acad Sci U S A. 2013;110(28):E2611–20.
Soltanpour S, Jouyban A. Solubility of acetaminophen and ibuprofen in polyethylene glycol 600, propylene glycol and water mixtures at 25°C. J Mol Liq. 2010;155(2):80–4.
Article CAS Google Scholar
Haglund BO. Solubility studies of polyethylene glycols in ethanol and water. Thermochimica Acta. 1987;114(1):97–102.
Petras D, Nothias LF, Quinn RA, Alexandrov T, Bandeira N, Bouslimani A, et al. Mass spectrometry-based visualization of molecules associated with human habitats. Anal Chem. 2016;88(22):10775–84.
Vazquez-Baeza Y, Pirrung M, Gonzalez A, Knight R. EMPeror: a tool for visualizing high-throughput microbial community data. Gigascience. 2013;2(1):16.
Wang M, Carver JJ, Phelan VV, Sanchez LM, Garg N, Peng Y, et al. Sharing and community curation of mass spectrometry data with Global Natural Products Social Molecular Networking. Nat Biotechnol. 2016;34(8):828–37.
Watrous J, Roach P, Alexandrov T, Heath BS, Yang JY, Kersten RD, et al. Mass spectral molecular networking of living microbial colonies. Proc Natl Acad Sci U S A. 2012;109(26):E1743–52.
Frank AM, Monroe ME, Shah AR, Carver JJ, Bandeira N, Moore RJ, et al. Spectral archives: extending spectral libraries to analyze both identified and unidentified spectra. Nat Methods. 2011;8(7):587–91.
Quinn RA, Nothias LF, Vining O, Meehan M, Esquenazi E, Dorrestein PC. Molecular networking as a drug discovery, drug metabolism, and precision medicine strategy. Trends Pharmacol Sci. 2017;38(2):143–54.
Luzzatto-Knaan T, Garg N, Wang M, Glukhov E, Peng Y, Ackermann G, et al. Digitizing mass spectrometry data to explore the chemical diversity and distribution of marine cyanobacteria and algae. Elife. 2017;6:e24214.
Melnik AV, da Silva RR, Hyde ER, Aksenov AA, Vargas F, Bouslimani A, et al. Coupling targeted and untargeted mass spectrometry for metabolome-microbiome-wide association studies of human fecal samples. Anal Chem. 2017;89(14):7549–59.
Sumner LW, Amberg A, Barrett D, Beale MH, Beger R, Daykin CA, et al. Proposed minimum reporting standards for chemical analysis Chemical Analysis Working Group (CAWG) Metabolomics Standards Initiative (MSI). Metabolomics. 2007;3(3):211–21.
Lozupone C, Knight R. UniFrac: a new phylogenetic method for comparing microbial communities. Appl Environ Microbiol. 2005;71(12):8228–35.
Caporaso JG, Lauber CL, Costello EK, Berg-Lyons D, Gonzalez A, Stombaugh J, et al. Moving pictures of the human microbiome. Genome Biol. 2011;12(5):R50.
Green BR. Chloroplast genomes of photosynthetic eukaryotes. Plant J. 2011;66(1):34–44.
Callewaert C, Kerckhof FM, Granitsiotis MS, Van Gele M, Van de Wiele T, Boon N. Characterization of Staphylococcus and Corynebacterium clusters in the human axillary region. PLoS One. 2013;8(8):e70538.
Callewaert C, Lambert J, Van de Wiele T. Towards a bacterial treatment for armpit malodour. Exp Dermatol. 2017;26(5):388–91.
Tripathi A, Melnik AV, Xue J, Poulsen O, Meehan MJ, Humphrey G, et al. Intermittent hypoxia and hypercapnia, a hallmark of obstructive sleep apnea, alters the gut microbiome and metabolome. mSystems. 2018;3(3):e00020-18.
Gower JC. Generalized procrustes analysis. Psychometrika [journal article]. 1975;40(1):33–51.
Decreau RA, Marson CM, Smith KE, Behan JM. Production of malodorous steroids from androsta-5,16-dienes and androsta-4,16-dienes by Corynebacteria and other human axillary bacteria. J Steroid Biochem Mol Biol. 2003;87(4–5):327–36.
Austin C, Ellis J. Microbial pathways leading to steroidal malodour in the axilla. J Steroid Biochem Mol Biol. 2003;87(1):105–10.
Lloyd-Price J, Mahurkar A, Rahnavard G, Crabtree J, Orvis J, Hall AB, et al. Strains, functions and dynamics in the expanded Human Microbiome Project. Nature. 2017;550(7674):61-6.
Kapono CA, Morton JT, Bouslimani A, Melnik AV, Orlinsky K, Knaan TL, et al. Creating a 3D microbial and chemical snapshot of a human habitat. Sci Rep. 2018;8(1):3669.
Clemente JC, Pehrsson EC, Blaser MJ, Sandhu K, Gao Z, Wang B, et al. The microbiome of uncontacted Amerindians. Sci Adv. 2015;1(3):e1500183.
Blaser MJ, Dominguez-Bello MG, Contreras M, Magris M, Hidalgo G, Estrada I, et al. Distinct cutaneous bacterial assemblages in a sampling of South American Amerindians and US residents. ISME J. 2013;7(1):85–95.
Nakatsuji T, Chen TH, Narala S, Chun KA, Two AM, Yun T, et al. Antimicrobials from human skin commensal bacteria protect against Staphylococcus aureus and are deficient in atopic dermatitis. Sci Transl Med 2017;9(378)eaah4680.
Hollands A, Gonzalez D, Leire E, Donald C, Gallo RL, Sanderson-Smith M, et al. A bacterial pathogen co-opts host plasmin to resist killing by cathelicidin antimicrobial peptides. J Biol Chem. 2012;287(49):40891–7.
Zirwas MJ, Moennich J. Antiperspirant and deodorant allergy: diagnosis and management. J Clin Aesthet Dermatol. 2008;1(3):38–43.
PubMed PubMed Central Google Scholar
Funk JO, Maibach HI. Propylene glycol dermatitis: re-evaluation of an old problem. Contact Dermatitis. 1994;31(4):236–41.
Lehmann R, Zhao X, Weigert C, Simon P, Fehrenbach E, Fritsche J, et al. Medium chain acylcarnitines dominate the metabolite pattern in humans under moderate intensity exercise and support lipid oxidation. PLoS One. 2010;5(7):e11519.
Hiatt WR, Regensteiner JG, Wolfel EE, Ruff L, Brass EP. Carnitine and acylcarnitine metabolism during exercise in humans. Dependence on skeletal muscle metabolic state. J Clin Invest. 1989;84(4):1167–73.
Fischbach MA, Segre JA. Signaling in host-associated microbial communities. Cell. 2016;164(6):1288–300.
Devlin AS, Fischbach MA. A biosynthetic pathway for a prominent class of microbiota-derived bile acids. Nat Chem Biol [Article]. 2015;11(9):685–90.
Ridlon JM, Kang DJ, Hylemon PB, Bajaj JS. Bile acids and the gut microbiome. Curr Opin Gastroenterol. 2014;30(3):332–8.
Humbert L, Maubert MA, Wolf C, Duboc H, Mahe M, Farabos D, et al. Bile acid profiling in human biological samples: comparison of extraction procedures and application to normal and cholestatic patients. J Chromatogr B Analyt Technol Biomed Life Sci. 2012;899:135–45.
Ghent CN, Bloomer JR. Itch in liver disease: facts and speculations. Yale J Biol Med. 1979;52(1):77–82.
CAS PubMed PubMed Central Google Scholar
Herndon JH Jr. Pathophysiology of pruritus associated with elevated bile acid levels in serum. Arch Intern Med. 1972;130(4):632–7.
Zapata HJ, Quagliarello VJ. The microbiota and microbiome in aging: potential implications in health and age-related diseases. J Am Geriatr Soc. 2015;63(4):776–81.
Kueneman JG, Woodhams DC, Harris R, Archer HM, Knight R, McKenzie VJ. Probiotic treatment restores protection against lethal fungal infection lost during amphibian captivity. Proc Biol Sci. 2016;283(1839):e20161553.
Woodhams DC, Brandt H, Baumgartner S, Kielgast J, Kupfer E, Tobler U, et al. Interacting symbionts and immunity in the amphibian skin mucosome predict disease risk and probiotic effectiveness. PLoS One. 2014;9(4):e96375.
Belkaid Y, Tamoutounour S. The influence of skin microorganisms on cutaneous immunity. Nat Rev Immunol. 2016;16(6):353–66.
Belkaid Y, Segre JA. Dialogue between skin microbiota and immunity. Science. 2014;346(6212):954–9.
Pluskal T, Castillo S, Villar-Briones A, Oresic M. MZmine 2: modular framework for processing, visualizing, and analyzing mass spectrometry-based molecular profile data. BMC Bioinformatics. 2010;11:395.
Dieterle F, Ross A, Schlotterbeck G, Senn H. Probabilistic quotient normalization as robust method to account for dilution of complex biological mixtures. Application in 1H NMR metabonomics. Anal Chem. 2006;78(13):4281–90.
Gilbert JA, Jansson JK, Knight R. The Earth Microbiome project: successes and aspirations. BMC Biol. 2014;12:69.
Caporaso JG, Lauber CL, Walters WA, Berg-Lyons D, Huntley J, Fierer N, et al. Ultra-high-throughput microbial community analysis on the Illumina HiSeq and MiSeq platforms. ISME J. 2012;6(8):1621–4.
Walters W, Hyde ER, Berg-Lyons D, Ackermann G, Humphrey G, Parada A, et al. Improved bacterial 16S rRNA gene (V4 and V4-5) and fungal internal transcribed spacer marker gene primers for microbial community surveys. mSystems. 2016;1(1):e00009-15.
Caporaso JG, Kuczynski J, Stombaugh J, Bittinger K, Bushman FD, Costello EK, et al. QIIME allows analysis of high-throughput community sequencing data. Nat Methods. 2010;7(5):335–6.
McDonald D, Price MN, Goodrich J, Nawrocki EP, DeSantis TZ, Probst A, et al. An improved Greengenes taxonomy with explicit ranks for ecological and evolutionary analyses of bacteria and archaea. ISME J. 2012;6(3):610–8.
Haferkamp I. The diverse members of the mitochondrial carrier family in plants. FEBS Lett. 2007;581(12):2375–9.
Burgess SA, Flint SH, Lindsay D, Cox MP, Biggs PJ. Insights into the Geobacillus stearothermophilus species based on phylogenomic principles. BMC Microbiol. 2017;17(1):140.
Goh KM, Gan HM, Chan KG, Chan GF, Shahar S, Chong CS, et al. Analysis of Anoxybacillus genomes from the aspects of lifestyle adaptations, prophage diversity, and carbohydrate metabolism. PLoS One. 2014;9(6):e90549.
Carvalhais LC, Dennis PG, Badri DV, Tyson GW, Vivanco JM, Schenk PM. Activation of the jasmonic acid plant defence pathway alters the composition of rhizosphere bacterial communities. PLoS One. 2013;8(2):e56457.
Barelli C, Albanese D, Donati C, Pindo M, Dallago C, Rovero F, et al. Habitat fragmentation is associated to gut microbiota diversity of an endangered primate: implications for conservation. Sci Rep. 2015;5:14862.
Gonzalez A, Navas-Molina JA, Kosciolek T, McDonald D, Vazquez-Baeza Y, Ackermann G, et al. Qiita: rapid, web-enabled microbiome meta-analysis. Nat Methods. 2018;15(10):796–8.
Gonzalez A, Vazquez-Baeza Y, Pettengill JB, Ottesen A, McDonald D, Knight R. Avoiding pandemic fears in the subway and conquering the platypus. mSystems. 2016;1(3):e00050-16.
Altschul SF, Gish W, Miller W, Myers EW, Lipman DJ. Basic local alignment search tool. J Mol Biol. 1990;215(3):403–10.
Wilkinson ADMaA. IUPAC. Compendium of chemical terminology, 2nd ed. (the "Gold Book": Blackwell Scientific Publications, Oxford 1997.
Smoot ME, Ono K, Ruscheinski J, Wang PL, Ideker T. Cytoscape 2.8: new features for data integration and network visualization. Bioinformatics. 2011;27(3):431–2.
Ward T, Larson J, Meulemans J, Hillmann B, Lynch J, Sidiropoulos D, et al. BugBase Predicts Organism Level Microbiome Phenotypes. bioRxiv. 2017;133462. https://doi.org/10.1101/133462 .
Langille MGI, Zaneveld J, Caporaso JG, McDonald D, Knights D, Reyes JA, et al. Predictive functional profiling of microbial communities using 16S rRNA marker gene sequences. Nat Biotech [Computational Biology]. 2013;31(9):814–21.
Download references
Acknowledgements
We thank all volunteers who were recruited in this study for their participation and Carla Porto for discussions regarding beauty products selected in this study. We further acknowledge Bruker for the support of the shared instrumentation infrastructure that enabled this work.
This work was partially supported by US National Institutes of Health (NIH) Grant. P.C.D. acknowledges funding from the European Union’s Horizon 2020 Programme (Grant 634402). A.B was supported by the National Institute of Justice Award 2015-DN-BX-K047. C.C. was supported by a fellowship of the Belgian American Educational Foundation and the Research Foundation Flanders. L.Z., J.K, and K.Z. acknowledge funding from the US National Institutes of Health under Grant No. AR071731. TLK was supported by Vaadia-BARD Postdoctoral Fellowship Award No. FI-494-13.
Availability of data and materials
The mass spectrometry data have been deposited in the MassIVE database (MSV000081582, MSV000081580 and MSV000081581). Molecular network parameters for MS/MS data collected from all body parts of 11 individuals during T0-T9 MSV000081582 are accessible here http://gnps.ucsd.edu/ProteoSAFe/status.jsp?task=284fc383e4c44c4db48912f01905f9c5 . Molecular network parameters for MS/MS data collected from armpits T0–T3 MSV000081582 and deodorant used by individual 1 and 3 MSV000081580 can be found here http://gnps.ucsd.edu/ProteoSAFe/status.jsp?task=f5325c3b278a46b29e8860ec5791d5ad and here http://gnps.ucsd.edu/ProteoSAFe/status.jsp?task=aaa1af68099d4c1a87e9a09f398fe253 , respectively. OTU tables can be found in Qiita ( qiita.ucsd.edu ) as study ID 10370, and sequences can be found in EBI under accession number EBI: ERP104625.
Author information
Amina Bouslimani and Ricardo da Silva contributed equally to this work.
Authors and Affiliations
Collaborative Mass Spectrometry Innovation Center, Skaggs School of Pharmacy and Pharmaceutical Sciences, San Diego, USA
Amina Bouslimani, Ricardo da Silva, Kathleen Dorrestein, Alexey V. Melnik, Tal Luzzatto-Knaan & Pieter C. Dorrestein
Department of Pediatrics, University of California, San Diego, La Jolla, CA, 92037, USA
Tomasz Kosciolek, Stefan Janssen, Chris Callewaert, Amnon Amir, Livia S. Zaramela, Ji-Nu Kim, Gregory Humphrey, Tara Schwartz, Karenina Sanders, Caitriona Brennan, Gail Ackermann, Daniel McDonald, Karsten Zengler, Rob Knight & Pieter C. Dorrestein
Department for Pediatric Oncology, Hematology and Clinical Immunology, University Children’s Hospital, Medical Faculty, Heinrich-Heine-University Düsseldorf, Düsseldorf, Germany
Stefan Janssen
Center for Microbial Ecology and Technology, Ghent University, 9000, Ghent, Belgium
Chris Callewaert
Center for Microbiome Innovation, University of California, San Diego, La Jolla, CA, 92307, USA
Karsten Zengler, Rob Knight & Pieter C. Dorrestein
Department of Bioengineering, University of California, San Diego, La Jolla, CA, 92093, USA
Karsten Zengler & Rob Knight
Department of Computer Science and Engineering, University of California, San Diego, La Jolla, CA, 92093, USA
Department of Pharmacology, University of California, San Diego, La Jolla, CA, 92037, USA
Pieter C. Dorrestein
You can also search for this author in PubMed Google Scholar
Contributions
AB and PCD contributed to the study and experimental design. AB, KD, and TLK contributed to the metabolite and microbial sample collection. AB contributed to the mass spectrometry data collection. AB, RS, and AVM contributed to the mass spectrometry data analysis. RS contributed to the metabolomics statistical analysis and microbial–molecular correlations. GH, TS, KS, and CB contributed to the 16S rRNA sequencing. AB and GA contributed to the metadata organization. TK, SJ, CC, AA, and DMD contributed to the microbial data analysis and statistics. LZ, JK, and KZ contributed to the additional data analysis. AB, PCD, and RK wrote the manuscript. All authors read and approved the final manuscript.
Corresponding authors
Correspondence to Rob Knight or Pieter C. Dorrestein .
Ethics declarations
Ethics approval and consent to participate.
All participants signed a written informed consent in accordance with the sampling procedure approved by the UCSD Institutional Review Board (Approval Number 161730).
Competing interests
Dorrestein is on the advisory board for SIRENAS, a company that aims to find therapeutics from ocean environments. There is no overlap between this research and the company. The other authors declare that they have no competing interests.
Publisher’s Note
Springer Nature remains neutral with regard to jurisdictional claims in published maps and institutional affiliations.
Additional files
Additional file 1:.
Figure S1. Beauty products ingredients persist on skin of participants. Figure S2. Beauty product application impacts the molecular and bacterial diversity on skin of 11 individuals while the chemical diversity from personal beauty products used by males and females on T0 is similar. Figure S3. Longitudinal impact of ceasing and resuming the use of beauty products on the molecular composition of the skin over time. Figure S4. Molecular networking to highlight MS/MS spectra found in each body part. Figure S5. Longitudinal abundance of bile acids and acylcarnitines in skin samples. Figure S6. Characterization of steroids in armpits samples. Figure S7. Characterization of bile acids in armpit samples. Figure S8. Characterization of Acylcarnitine family members in skin samples. Figure S9. Beauty products applied at one body part might affect other areas of the body, while specific products determine stability versus variability of microflora at each body site. Figure S10. Representation of Gram-positive bacteria over time and the molecular features from the shampoo detected on feet. Figure S11. Procrustes analysis to correlate the skin microbiome and metabolome over time. Figure S12. Correlation between specific molecules and bacteria that change over time in armpits of individual 11. Figure S13. Representation of the number of samples that were removed (gray) and those retained (blue) after rarefaction at 10,000 threshold. (DOCX 1140 kb)
Additional file 2:
Table S1. List of personal (T0 and T7–9) beauty products and their frequency of use. (XLSX 30 kb)
Additional file 3:
Table S2. List of ingredients of common beauty products used during T4–T6. (PDF 207 kb)
Additional file 4:
Table S3. Mzmine feature finding and crop filtering parameters. (XLSX 4 kb)
Additional file 5:
Table S4. Feature table for statistical analysis with blank filtering and total ion current normalization. (CSV 150242 kb)
Additional file 6:
Table S5. Feature table for individual feature abundance in armpits. (XLSX 379 kb)
Additional file 7:
Table S6. Feature table for Calour analysis. (CSV 91651 kb)
Additional file 8:
Table S7. Metadata for Calour analysis. (TXT 129 kb)
Additional file 9:
Table S8. feature table with Probabilistic quotient normalization for molecular–microbial analysis. (ZIP 29557 kb)
Additional file 10:
Table S9. OTU table rarefied to 10,000 sequences per sample. (BIOM 9493 kb)
Additional file 11:
Table S10. 16S rRNA sequencing read counts per sample. (TSV 2949 kb)
Rights and permissions
Open Access This article is distributed under the terms of the Creative Commons Attribution 4.0 International License ( http://creativecommons.org/licenses/by/4.0/ ), which permits unrestricted use, distribution, and reproduction in any medium, provided you give appropriate credit to the original author(s) and the source, provide a link to the Creative Commons license, and indicate if changes were made. The Creative Commons Public Domain Dedication waiver ( http://creativecommons.org/publicdomain/zero/1.0/ ) applies to the data made available in this article, unless otherwise stated.
Reprints and permissions
About this article
Cite this article.
Bouslimani, A., da Silva, R., Kosciolek, T. et al. The impact of skin care products on skin chemistry and microbiome dynamics. BMC Biol 17 , 47 (2019). https://doi.org/10.1186/s12915-019-0660-6
Download citation
Received : 20 February 2019
Accepted : 30 April 2019
Published : 12 June 2019
DOI : https://doi.org/10.1186/s12915-019-0660-6
Share this article
Anyone you share the following link with will be able to read this content:
Sorry, a shareable link is not currently available for this article.
Provided by the Springer Nature SharedIt content-sharing initiative
- Skin care products
- Mass spectrometry
- Metabolomics
- 16S rRNA sequencing
BMC Biology
ISSN: 1741-7007
- Submission enquiries: [email protected]
- General enquiries: [email protected]
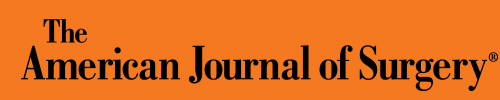
WORLD JOURNAL OF COSMETOLOGY
& AESTHETIC MEDICINE

List of Issues
Supports Open Access

World Journal of Cosmetology and Aesthetic Medicine is an international open access journal the basis of theoretical research and clinical application of advanced academic journals focused, senior-oriented professionals, Medical Aesthetics and Cosmetology-based academic journals. Areas of expertise include cosmetic surgery magazine, cosmetic surgery, cosmetic dermatology, cosmetic dentistry and cosmetic medicine. Contents: cosmetic surgery, cosmetic dermatology, cosmetic dentistry, cosmetic medicine, cosmetic medicine, physical beauty, cosmetic drugs, cosmetic and beauty care, medicine research and application articles. Reported in related areas of new technology, new materials, new progress. Selected other clinical disciplines but also in the field of aesthetic problems; to strengthen management and professional ethics of medical and cosmetic aspects of the experience; guide the occupational health and aesthetic cultivation of aesthetic education and other topics. Part with: treatise, experimental research, medical aesthetics, lessons learned, case reports, technical innovation, review and so on.
The World Journal of Cosmetology and Aesthetic Medicine is published by Boston Science Publishing has always been at the forefront in crafting awareness among, Research Scientist, Academic Professors, Authors, Librarians and Graduate students how would like to showcase their work..
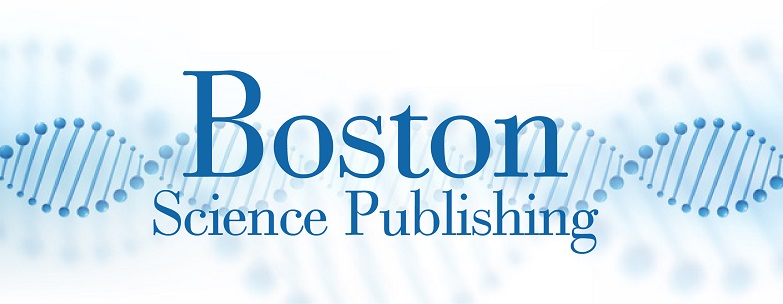
Journal Information
Current issue.
New Technique for Reducing and Modeling Nasolabial Fat and Jowls: Lipoestructuración
FELICIDADE, Sidnei Iensen 1 , PhD; LOPEZ, Coreli Coromoto Mendoza 2 , MD
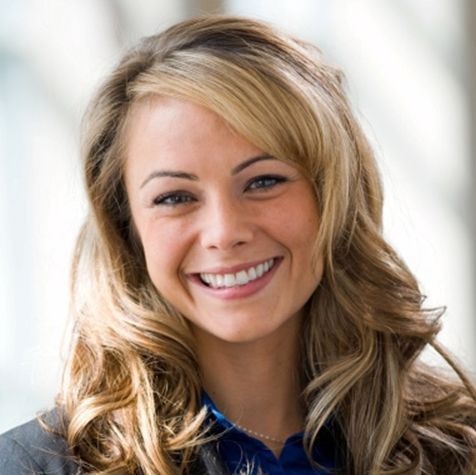
Laura A. Monson
These journal metrics provide authors with extra insight into the journals and help them in their journal selection process when submitting an article for publication.
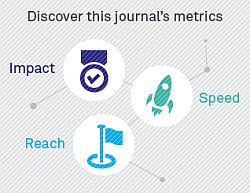
ARTICLES PROMOTION
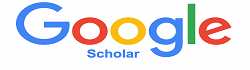
Stay Up to Date

- Browse All Articles
- Newsletter Sign-Up
No results found in Working Knowledge
- Were any results found in one of the other content buckets on the left?
- Try removing some search filters.
- Use different search filters.
BU Study Shows a Correlation between Social Media Use and Desire for Cosmetic Procedures
The more time you spend on image-led social media, like instagram and snapchat, the more likely you are to want to alter your appearance.
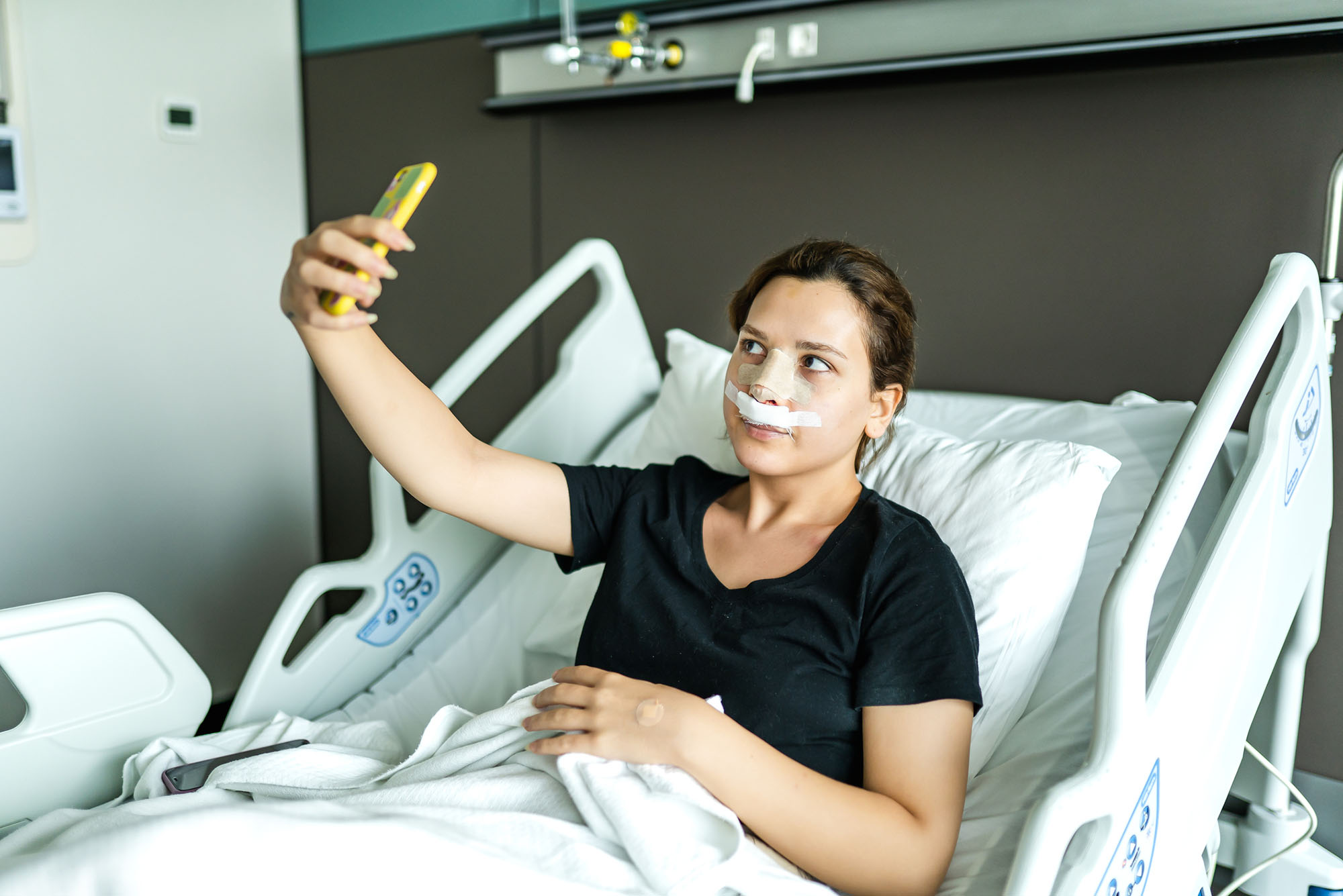
Have you ever considered getting a cosmetic procedure? If so, new research from BU dermatologists suggests your social media activity could play a part in your decision-making. Photo via iStock/Su Arslanoglu
Alene Bouranova
It’s a familiar pattern: you open your social media app of choice and end up sucked into a digital wormhole, mindlessly scrolling while the real world fades away. Maybe you even decide to post something, and pick out a filter to apply to your latest selfie or vacation photo. Or maybe you run your pic through an app like Facetune, tweaking your appearance to look your best.
Your photo then becomes one of the countless altered images coursing through social media users’ feeds, subtly shifting the goalposts for conventions of attractiveness. The question is: What effect does that have on our perceptions of ourselves and our willingness to make more permanent changes to our appearance?
A new study from dermatologists at the Boston University Chobanian & Avedisian School of Medicine demonstrates a suspected trend: the more time you spend on social media, scrolling past endless images of perfectly sculpted faces and bodies, the more likely you are to want to undergo a cosmetic procedure.
The study comes at a time when it’s easier than ever to go under the knife (or needle) to alter your appearance. Elective procedures—non-medically necessary dermal fillers, Botox, chemical peels, laser treatments, implants, plastic surgery—have become more accessible in recent years, in part due to developments in cosmetic technology. And, with more and more celebrities being open about their look-changing procedures—like actress Megan Fox on Alex Cooper’s (COM’17) Call Her Daddy podcast —they’re also much less taboo.
The BU researchers set out to understand how social media influences users’ perceptions of beauty, and if popular apps inadvertently encourage people to alter their appearance. They polled 175 individuals at an outpatient dermatology clinic from 2019 to 2022 about their social media usage, their perception of cosmetic procedures, and their personal desire to undergo cosmetic procedures. The study also split respondents into two groups—pre-pandemic and post-pandemic—to analyze whether a recent national rise in cosmetic dermatology was driven by COVID-era changes in social media and video conferencing use. The findings were published in the Journal of Clinical and Aesthetic Dermatology .
The results showed that spending time on image-led platforms like Instagram and Snapchat—and, in particular, adding filters or using photo-editing apps before sharing photos—strongly correlated with respondents’ desire to undergo a cosmetic procedure. The researchers also found that following influencers and celebrities on social media made respondents significantly more likely to want to undergo cosmetic work, as did following accounts that highlight cosmetic procedures (such as those that analyze before and after pictures). The study noted a major bump among respondents from the post-pandemic group who followed cosmetic procedure–related accounts online and indicated a desire to undergo work of their own.
“One of the most significant findings was that many more people post-COVID had thought about having a cosmetic procedure done, or had even discussed it with a dermatologist or a physician, and believed that doing so would help their self-esteem,” says Neelam Vashi , a BU medical school associate professor of dermatology and corresponding author on the study.
One of the most significant findings was that many more people post-COVID had thought about having a cosmetic procedure done, or had even discussed it with a dermatologist or a physician, and believed that doing so would help their self-esteem. Neelam Vashi
That doesn’t mean social media is directly responsible for patients going out and seeking treatment, Vashi says. But a user who was already considering pursuing a treatment—say, lip injections for fuller lips or buccal fat removal for more pronounced cheekbones—is more likely to book an appointment as a result of targeted exposure to procedures online.
The findings weren’t necessarily surprising to Vashi, founder of the BU Center for Ethnic Skin and director of the BU Cosmetic and Laser Center at Boston Medical Center, the University’s primary teaching hospital. “Much of my research revolves around understanding the intricacies of beauty perception, and what defines beauty standards,” she says. “In the logic of social media, the use of filters has completely changed our perception of beauty and what can be achieved.”
And it’s not just social media driving the results. The rise of video conferencing during COVID lockdowns also likely contributed to patients’ increasing desire to get work done, the researchers concluded. “Even on Zoom, there’s a feature where you can blur your appearance and remove any blemishes,” says Vashi, who notes an uptick in her patients asking for procedures to even their skin tones.
Self-esteem is at the core of the study, according to Vashi. In our increasingly visual world—and one in which everything we see online is potentially edited or AI-generated—it can be demoralizing when your appearance doesn’t match up with the “ideals” presented to you online. Even when it’s your own face you’re comparing yourself to: in the study, the research team cites Vashi’s prior writings on “Snapchat dysmorphia,” which describes patients dissatisfied by not looking like the filtered versions of themselves they post online.
In the past, Vashi’s patients would sometimes bring in photographs of celebrities they wanted to emulate. “Now, when people are able to filter their own face and make it look more beautiful—even the skin tone, angulate the chin, raise the cheekbones, enlarge the eyes—they bring in these images of themselves that have become very realistic to them because it’s just a beautified version of their own face,” she says.
Of course, Vashi adds, there’s nothing wrong with wanting to improve your appearance—that’s why we do things like workout, wear makeup, buy new clothes, and myriad other improvement-focused activities. It only becomes concerning when patients seek out cosmetic changes as a fix-all Band-Aid for their self-esteem issues, or become fixated on unrealistic expectations for themselves, she says.
Going forward, Vashi hopes the study motivates dermatologists and other providers to further check in with patients about their social media usage and why they might be seeking treatments. She also hopes it inspires social media users to be mindful of how they use the internet, and to step away from their screen if they notice their self-esteem taking a hit. Parents, too, should monitor what their children are consuming online, and should be prepared to have conversations about how that content can influence how they feel about themselves.
Zoom, social media—“they’re not going anywhere,” Vashi says. “In looking at ourselves and comparing it to what we see on screens, it’s really going to take an effort by all parties to keep a healthy outlook on what we see online.”
Explore Related Topics:
- Social Media
- Share this story
- 0 Comments Add
Writer/Editor Twitter Profile
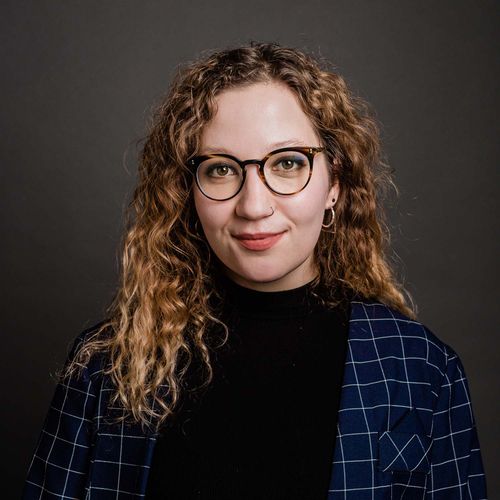
Alene Bouranova is a Pacific Northwest native and a BU alum (COM’16). After earning a BS in journalism, she spent four years at Boston magazine writing, copyediting, and managing production for all publications. These days, she covers campus happenings, current events, and more for BU Today . Fun fact: she’s still using her Terrier card from 2013. When she’s not writing about campus, she’s trying to lose her Terrier card so BU will give her a new one. She lives in Cambridge with her plants. Profile
Alene Bouranova can be reached at [email protected]
Comments & Discussion
Boston University moderates comments to facilitate an informed, substantive, civil conversation. Abusive, profane, self-promotional, misleading, incoherent or off-topic comments will be rejected. Moderators are staffed during regular business hours (EST) and can only accept comments written in English. Statistics or facts must include a citation or a link to the citation.
Post a comment. Cancel reply
Your email address will not be published. Required fields are marked *
Latest from The Brink
Covid-19 photo contest winners capture moments of joy, sorrow, meaning in crisis, how high-level lawsuits are disrupting climate change policies, the h5n1 bird flu is a growing threat for farm animals and humans—how serious is it, why is a bu researcher so fascinated with the diets of dung beetles, we are underestimating the health harms of climate disasters, three bu researchers elected aaas fellows, should people be fined for sleeping outside, secrets of ancient egyptian nile valley settlements found in forgotten treasure, bu electrical engineer vivek goyal named a 2024 guggenheim fellow, not having job flexibility or security can leave workers feeling depressed, anxious, and hopeless, can the bias in algorithms help us see our own, do immigrants and immigration help the economy, how do people carry such heavy loads on their heads, do alcohol ads promote underage drinking, how worried should we be about us measles outbreaks, stunning new image shows black hole’s immensely powerful magnetic field, it’s not just a pharmacy—walgreens and cvs closures can exacerbate health inequities, how does science misinformation affect americans from underrepresented communities, what causes osteoarthritis bu researchers win $46 million grant to pursue answers and find new treatments.
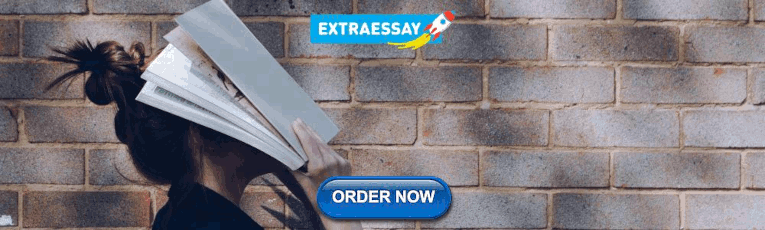
IMAGES
VIDEO
COMMENTS
Benefits of publishing with International Journal of Cosmetic Science: • Extensive readership, with 2,500 society members accessing the journal. • Fully indexed in ISI and MedLine. • Free Access to all Reviews. • Accepted Articles; Accepted, unedited articles published online for future issues.
The literature review indicates that beauty salons may also be suitable places for increasing the awareness about pregnancy, motherhood and child health. 6,9,19,25 One of the studies showed that pregnancy and motherhood were the most frequently discussed health-related topics in beauty salons. 25 However, the research done by Ahlers-Schmidt et ...
"L'Oréal's research group has a strong interest in leveraging regenerative medicine concepts and advances to create the future of beauty, which we believe will be regenerative," Bouez says.
1. Introduction. Nanotechnology and nanodelivery systems are innovative areas of science that comprise the design, characterization, manufacturing, and application of materials, devices, and systems at the nanoscale level (1-100 nm). Nanotechnology, being recognized as one of the revolutionizing technologies, is extensively studied in the ...
Explore the latest full-text research PDFs, articles, conference papers, preprints and more on COSMETOLOGY. Find methods information, sources, references or conduct a literature review on COSMETOLOGY
A beauty salon, as a place of social interaction, may constitute an area of implementation of pro-health educational programs. Flow chart of the articles selection process. Summary of the reviewed ...
Background: Contemporary cosmetology, apart from beautifying and caring for the human body, deals also with prevention aimed at maintaining health and physical fitness as long as possible. The profession of a cosmetologist so understood is closely related to the modern concept of health promotion, the part of which is health education.
The efficacy of topical synthetic Vitamin A or retinoids—in various forms such as tretinoin, adapalene, and tazarotene—is evidence-based, and the cosmetic benefits of these prescription retinoids are well supported by ample research. 2, 3 Tretinoin induces production of type I and II procollagen in skin and reduces collagen breakdown by ...
Introduction. Rosacea is a chronic skin disease usually occurring only in the facial area. Clinically, it manifests itself with an erythema, telangiectasias, and papules and pustules, while histopathologically - it is an inflammatory condition with a perivascular infiltrate, dilated blood vessels, lymphoedema, hyperplasia of sebaceous glands, and disorders of connective tissue structures ...
Many people in the scientific community don't appreciate the breadth and depth of the serious science that happens in beauty. The Estée Lauder Companies' R&D teams are constantly looking at ...
Abstract. This review paper is based on the use of nanotechnology in the world of cosmetics and cosmeceuticals by increasing the effectiveness of the product. Nanotechnology has been considered to be a novel innovation of the period in different fields of science. The different types of nanocarriers that are used in cosmetics such as cubosomes ...
Sustainability is a 'hot' subject this millennium. While reviewing sustainability related to the cosmetic industry, we observed that there is an enormous amount of information in research and review papers, scientific and non-scientific (beauty, well-being, etc.) websites, online articles, and periodicals, etc.
Cosmetics. , Volume 9, Issue 1 (February 2022) - 26 articles. Cover Story ( view full-size image ): Red palm fruits (Elaeis guineensis) extract contains high levels of vitamin E, b-carotene, and palmitic acid has strong antioxidant activity and is suitable for skin care. Solid lipid nanoparticles (SLNs) were employed to deliver active extract ...
Biocosmetics are cosmetic products made from 100% natural ingredients derived from plants, animals, microbes, enzymes, insects, and organic crops that are free of pesticides and chemical fertilizers and used for topical skin, hair, face, and oral care (Vandamme 2001; Novak et al. 2014).Most of the conventional skin-care cosmetic formulations use petroleum or mineral oil-derived ingredients ...
Cosmetics. Cosmetics is an international, scientific, peer-reviewed, open access journal on the science and technology of cosmetics published bimonthly online by MDPI. Open Access — free for readers, with article processing charges (APC) paid by authors or their institutions. High Visibility: indexed within Scopus, ESCI (Web of Science ...
Achievement goal orientation, motivation learning, beauty education, post‐COVID‐19, results in 40 asymptomatic academic achievements, qualification evaluation, 39 papers, excluding 1 expert articles (protocol/method papers without results, 1 expert articles) have been finalized. This was shown using PRISMA diagram (Figure 1 ).
Scientists 3D-Print Hair Follicles in Lab-Grown Skin. Nov. 15, 2023 — Scientists have 3D-printed hair follicles in human skin tissue cultured in the lab. This marks the first time researchers ...
Background Use of skin personal care products on a regular basis is nearly ubiquitous, but their effects on molecular and microbial diversity of the skin are unknown. We evaluated the impact of four beauty products (a facial lotion, a moisturizer, a foot powder, and a deodorant) on 11 volunteers over 9 weeks. Results Mass spectrometry and 16S rRNA inventories of the skin revealed decreases in ...
Explore the latest full-text research PDFs, articles, conference papers, preprints and more on COSMETOLOGY. Find methods information, sources, references or conduct a literature review on COSMETOLOGY
An Online Survey to Estimate the Knowledge, Attitude, and Practice among People in Odisha on Proper Drug Disposal Methods along with their Opinion on the Use of E-pharmacy and Drones as Future Dispensing Models for Medicines. View More. World Journal of Cosmetology and Aesthetic Medicine is an international open access journal the basis of ...
HBS Cases: Beauty Entrepreneur Madam Walker. by Martha Lagace. She may have been the first self-made African American millionaire. Born of emancipated slaves, Madam C.J. Walker traveled from the cotton fields to business fame as a purveyor of hair-care products that offered beauty and dignity.
Multi-disciplinary database; full text of articles from over 5,300 journals, magazines and newspapers, plus image collections. Learn how to start, finance, or manage your small business. Includes sample business plans, how to guides, articles, and more.
According to the UK Home Office ( 12 ), in the year 2016, 48.6% of the animal tests in medical research were conducted for genetically oriented studies. Moreover, 28.5% of the medical research involving animal testing was for basic biological research, 13.5% was for regulatory. testing, 8.6% was for translating research from animals to humans ...
Global attention to nail health and esthetic appearance. Throughout history, women have enhanced their beauty with nail cosmetics. The use of nail cosmetics dates to 5000 BC when women in India, China, and Egypt used henna to dye their fingernails (Shafer, 1993, Tsatalis et al., 2018).Currently, 85% to 90% of women worldwide use nail care products (Goldstein Research, 2018).
The BU researchers set out to understand how social media influences users' perceptions of beauty, and if popular apps inadvertently encourage people to alter their appearance. They polled 175 individuals at an outpatient dermatology clinic from 2019 to 2022 about their social media usage, their perception of cosmetic procedures, and their ...