
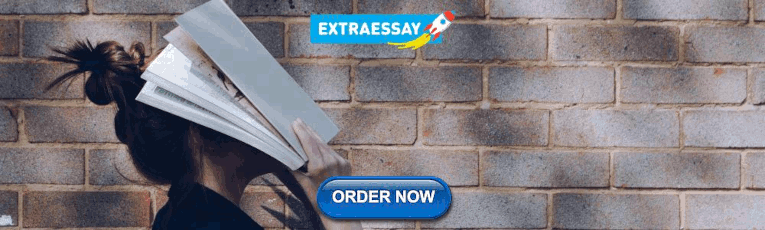
How plants can generate electricity to power LED light bulbs
Sustainable energy sources, which are pollution free and environmentally friendly, are one of the key challenges of world's future society. The interdisciplinary team of roboticists and biologists at IIT-Istituto Italiano di Tecnologia in Pontedera (Pisa, Italy), found that living plants can help with electricity. Fabian Meder, Barbara Mazzolai and their coworkers at IIT discovered that living plants are literally "green" power source, which may become one of future's electricity supplies that perfectly integrates in natural environments and is accessible all over the world. Researchers discovered that plants can generate, by a single leaf, more than 150 Volts, enough to simultaneously power 100 LED light bulbs. Researchers also showed that an "hybrid tree" made of natural and artificial leaves can act as an innovative "green" electrical generator converting wind into electricity.
Results are published on Advanced Functional Materials .
The research team is based at Center for Micro-Bio Robotics (CMBR) of IIT in Pontedera (Pisa, Italy), coordinated by Barbara Mazzolai, and their goal is to perform advanced research and to develop innovative methodologies, robotic technologies and new materials, inspired by the natural world. Bio-inspired approaches can therefore help to develop robots and technologies that are more suitable for unstructured environments than today's solutions. In 2012 Barbara Mazzolai coordinated the EU funded project Plantoid, which brought to the realization of the first plant robot in the world. In this last study, the research team studied plants and showed that leaves can create electricity when they are touched by a distinct material or by the wind.
Certain leaf structures are capable to convert mechanical forces applied at the leaf surface into electrical energy, because of the specific composition that most plant leaves naturally provide. In detail, the leaf is able to gather electric charges on its surface due to a process called contact electrification. These charges are then immediately transmitted into the inner plant tissue. The plant tissue acts similar to a "cable" and transports the generated electricity to other parts of the plant. Hence, by simply connecting a "plug" to the plant stem, the electricity generated can be harvested and used to power electronic devices. IIT's researchers show that the voltage generated by a single leaf may reach to more than 150 Volts, enough to simultaneously power 100 LED light bulbs each time the leaf is touched.
In the article, researchers additionally describe for the first time how this effect can be used to convert wind into electricity by plants. Therefore, researchers modified a Nerum oleander tree with artificial leaves that touch the natural N. oleander leaves. When wind blows into the plant and moves the leaves, the "hybrid tree" produces electricity. The electricity generated increases the more leaves are touched. Consequently, it can be easily up-scaled by exploiting the whole surface of the foliage of a tree or even a forest.
The study is a first essential step for a new project that Barbara Mazzolai will coordinate in 2019, the European-funded project Growbot whose aim is to realize bioinspired robots that implement plant-like growing motions. The new robots will be then partly powered by the new plant-derived energy source, showing that plants may become one of future's electricity supplies, accessible all over the world.
- Endangered Plants
- Electricity
- Energy Technology
- Wind Energy
- Energy and Resources
- Power station
- Electricity generation
- Wind turbine
- Electric power transmission
- Hydroelectricity
- Electrical engineering
Story Source:
Materials provided by Istituto Italiano di Tecnologia - IIT . Note: Content may be edited for style and length.
Journal Reference :
- Fabian Meder, Indrek Must, Ali Sadeghi, Alessio Mondini, Carlo Filippeschi, Lucia Beccai, Virgilio Mattoli, Pasqualantonio Pingue, Barbara Mazzolai. Energy Conversion at the Cuticle of Living Plants . Advanced Functional Materials , 2018; 1806689 DOI: 10.1002/adfm.201806689
Cite This Page :
Explore More
- This Alloy Is Kinky
- Giant Galactic Explosion: Galaxy Pollution
- Flare Erupting Around a Black Hole
- Two Species Interbreeding Created New Butterfly
- Warming Antarctic Deep-Sea and Sea Level Rise
- Octopus Inspires New Suction Mechanism for ...
- Cities Sinking: Urban Populations at Risk
- Puzzle Solved About Ancient Galaxy
- How 3D Printers Can Give Robots a Soft Touch
- Combo of Multiple Health Stressors Harming Bees
Trending Topics
Strange & offbeat.
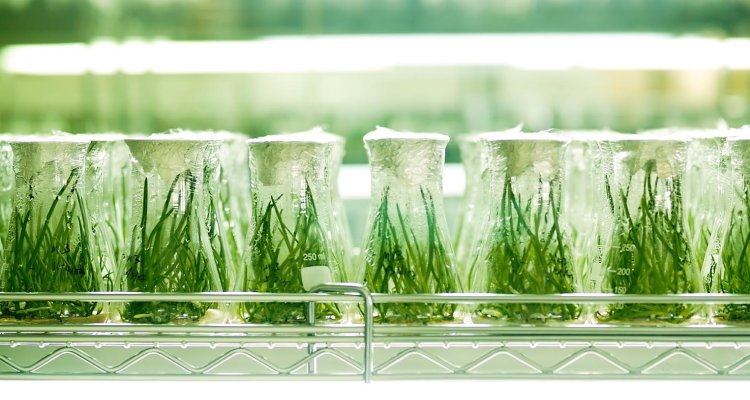
Plants create energy
Experiments have proven that plants can serve as a source of clean and renewable energy.
As part of the European project PlantPower, researchers are studying whether this technology is suitable for large-scale applications.They are also examining the technical feasibility and the economic profitability.
Scientists all over the world are looking for alternatives to fossil fuels. Plant materials are already used as an energy source through bio-fermentation. Now it appears that living plants can also contribute to energy production. In a plant microbial fuel cell, living plants work together with micro-organisms to create electricity. This results in clean and renewable energy, while the plant remains alive. The first practical tests have been very promising. In projects supported by the EU and Agentschap NL (an agency of the Dutch Ministry of Economic Affairs), European and Dutch institutes, universities, and businesses are cooperating to gain more knowledge about this technology and its applications.
Plant microbial fuel cell
The plant microbial fuel cell , developed by Bert Hamelers of the Sub-department of Environmental Technology at Wageningen University, was first described in 2008. The cell is based on the following principle: With the aid of sunlight, plants convert CO2 into organic compounds (photosynthesis). The plant uses some of the compounds which arise in this way for its own growth, while the remainder is eliminated through the roots. Micro-organisms which are naturally found in the ground around the roots of plants break down these organic compounds. This process causes electrons to be released. It is possible to gather these electrons with an electrode and use them to generate electricity. This system is capable of supplying green energy 24 hours a day, seven days a week. The direct current which is produced in this manner has a low voltage (1V) and as such is not dangerous for animals or plants.
Wageningen scientists
Some of the topics which the PlantPower researchers are examining include the processes in the plant, the micro-organisms in the rhizosphere (root zone), the existing energy losses, and the most suitable materials for a plant microbial fuel cell. Scientists from Wageningen have a prominent role at PlantPower. Bert Hamelers, Assistant Professor at the Sub-department of Environmental Technology, acts as project coordinator. Staff members of his sub-department focus on combining the various constituent processes into a viable system in the plant microbial fuel cell. Researchers from Wageningen UR Greenhouse Horticulture (website in Dutch) are primarily studying the substances eliminated by the plants and the applicability of the cell for food crops such as tomato plants.
Producing electricity
Links (some links in dutch):.
- Blog Plant-e
- Agentschap NL
- Universiteit Gent
- Maris Projects BV
- De Ruiter Seeds BV
- Universite de Rennes
- Microbial Fuel Cells
- Wilhelm Eisenhuth GmbH KG
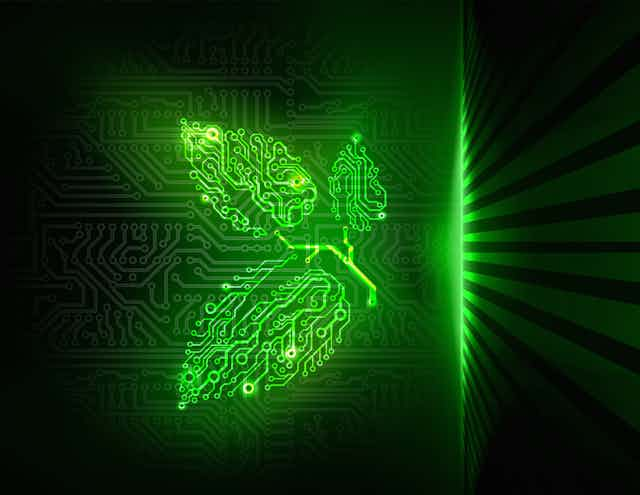
Scientists create electric circuits inside plants
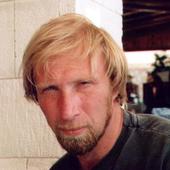
Senior Lecturer in Plant Biochemistry, University of Westminster
Disclosure statement
Stuart Thompson has received funding from MAFF and the Nuffield Foundation.
University of Westminster provides funding as a member of The Conversation UK.
View all partners
Plants power life on Earth. They are the original food source supplying energy to almost all living organisms and the basis of the fossil fuels that feed the power demands of the modern world. But burning the remnants of long-dead forests is changing the world in dangerous ways. Can we better harness the power of living plants today?
One way might be to turn plants into natural solar power stations that could convert sunlight into energy far more efficiently. To do this, we’d need a way of getting the energy out in the form of electricity. One company has found a way to harvest electrons deposited by plants into the soil beneath them. But new research from Finland looks at tapping plants’ energy directly by turning their internal structures into electric circuits.
Plants contain water-filled tubes called “xylem elements” that carry water from their roots to their leaves. The water flow also carries and distributes dissolved nutrients and other things such as chemical signals. The Finnish researchers, whose work is published in PNAS, developed a chemical that was fed into a rose cutting to form a solid material that could carry and store electricity.
Previous experiments have used a chemical called PEDOT to form conducting wires in the xylem, but it didn’t penetrate further into the plant. For the new research, they designed a molecule called ETE-S that forms similar electrical conductors but can also be carried wherever the stream of water travelling though the xylem goes.
This flow is driven by the attraction between water molecules. When water in a leaf evaporates, it pulls on the chain of molecules left behind, dragging water up through the plant all the way from the roots. You can see this for yourself by placing a plant cutting in food colouring and watching the colour move up through the xylem. The researchers’ method was so similar to the food colouring experiment that they could see where in the plant their electrical conductor had travelled to from its colour.
The result was a complex electronic network permeating the leaves and petals, surrounding their cells and replicating their pattern. The wires that formed conducted electricity up to a hundred times better than those made from PEDOT and could also store electrical energy in the same way as an electronic component called a capacitor.
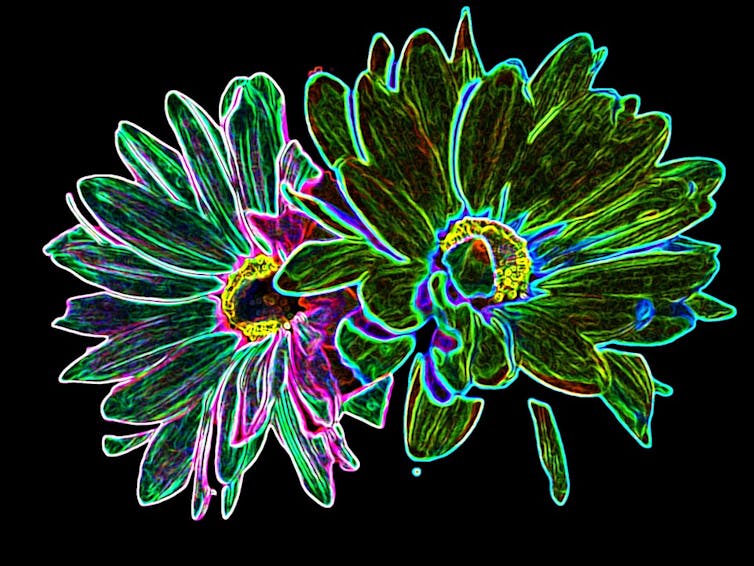
How well these electrical networks formed surprised even their developers. This seems to be because when the roses were treated with ETE-S, they produced the same reactive chemicals that they use to kill invading microorganisms. These chemicals made the formation of the solid electrical conductor work much better inside the plant than when it was tested in the lab.
There are still challenges before this discovery can achieve its full potential. Perhaps most importantly, they need to find a way of getting ETE-S (or some further improved chemical) into intact, living plants. But the creation of “e-plants”, that is plants with integrated electronic circuits, now looks much closer.
So how could e-plants be used? The most exciting possibility will be if we can combine e-plant electrical storage and circuitry with some way to directly tap photosynthetic energy, creating a literally green energy source.
But the technology could also help us better understand regular plants. Plants do not have a nervous system as animals do, but they do use electrical signals both to control individual cells and two carry messages between different parts of the plant. Perhaps the most spectacular example of this is in the Venus flytrap, in which the snapping mechanism is activated by an electrical impulse .
Building electrical circuits into plants will allow us to listen into these messages more easily. Perhaps when we understand their “language” better, we will then be able to send instructions to the plant. For example turning on its defence systems if we know that it is at risk of disease.
Perhaps we could create electronic plants that function like machines. If a crop could tell us if it has too little water or fertiliser, or is being attacked by insects, we could move resources to where they are most needed, improving farming efficiency. Maybe one day you could even use the technology to adjust a flower’s fragrance to match your mood.
- Biotechnology
- Solar power
- Bio engineering
- Solar energy
- Organic electronics
- Plant biotechnology

Senior Lecturer - Earth System Science

Associate Director, Operational Planning

Sydney Horizon Educators (Identified)

Deputy Social Media Producer

Associate Professor, Occupational Therapy
- You are here:
- American Chemical Society
- Discover Chemistry
Producing ‘green’ energy — literally — from living plant ‘bio-solar cells’
FOR IMMEDIATE RELEASE
“Self-Enclosed Bio-Photoelectrochemical Cell in Succulent Plants” ACS Applied Materials & Interfaces
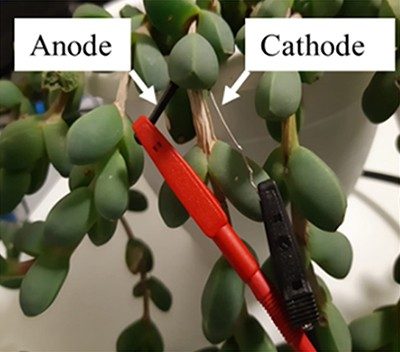
Though plants can serve as a source of food, oxygen and décor, they’re not often considered to be a good source of electricity. But by collecting electrons naturally transported within plant cells, scientists can generate electricity as part of a “green,” biological solar cell. Now, researchers reporting in ACS Applied Materials & Interfaces have, for the first time, used a succulent plant to create a living “bio-solar cell” that runs on photosynthesis.
In all living cells, from bacteria and fungi to plants and animals, electrons are shuttled around as part of natural, biochemical processes. But if electrodes are present, the cells can actually generate electricity that can be used externally. Previous researchers have created fuel cells in this way with bacteria, but the microbes had to be constantly fed. Instead, scientists, including Noam Adir’s team, have turned to photosynthesis to generate current. During this process, light drives a flow of electrons from water that ultimately results in the generation of oxygen and sugar. This means that living photosynthetic cells are constantly producing a flow of electrons that can be pulled away as a “photocurrent” and used to power an external circuit, just like a solar cell.
Certain plants — like the succulents found in arid environments — have thick cuticles to keep water and nutrients within their leaves. Yaniv Shlosberg, Gadi Schuster and Adir wanted to test, for the first time, whether photosynthesis in succulents could create power for living solar cells using their internal water and nutrients as the electrolyte solution of an electrochemical cell.
The researchers created a living solar cell using the succulent Corpuscularia lehmannii , also called the “ice plant.” They inserted an iron anode and platinum cathode into one of the plant’s leaves and found that its voltage was 0.28V. When connected into a circuit, it produced up to 20 µA/cm 2 of photocurrent density, when exposed to light and could continue producing current for over a day. Though these numbers are less than that of a traditional alkaline battery, they are representative of just a single leaf. Previous studies on similar organic devices suggest that connecting multiple leaves in series could increase the voltage. The team specifically designed the living solar cell so that protons within the internal leaf solution could be combined to form hydrogen gas at the cathode, and this hydrogen could be collected and used in other applications. The researchers say that their method could enable the development of future sustainable, multifunctional green energy technologies.
The authors acknowledge funding from a “Nevet” grant from the Grand Technion Energy Program (GTEP) and a Technion VPR Berman Grant for Energy Research and support from the Technion’s Hydrogen Technologies Research Laboratory (HTRL).
The American Chemical Society (ACS) is a nonprofit organization chartered by the U.S. Congress. ACS’ mission is to advance the broader chemistry enterprise and its practitioners for the benefit of Earth and all its people. The Society is a global leader in promoting excellence in science education and providing access to chemistry-related information and research through its multiple research solutions, peer-reviewed journals, scientific conferences, eBooks and weekly news periodical Chemical & Engineering News . ACS journals are among the most cited, most trusted and most read within the scientific literature; however, ACS itself does not conduct chemical research. As a leader in scientific information solutions, its CAS division partners with global innovators to accelerate breakthroughs by curating, connecting and analyzing the world’s scientific knowledge. ACS’ main offices are in Washington, D.C., and Columbus, Ohio.
To automatically receive press releases from the American Chemical Society, contact newsroom@acs.org .
Note: ACS does not conduct research, but publishes and publicizes peer-reviewed scientific studies.
Media Contact
ACS Newsroom newsroom@acs.org
Related Content
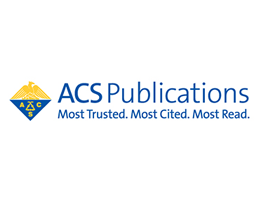
More From This Series

Accept & Close The ACS takes your privacy seriously as it relates to cookies. We use cookies to remember users, better understand ways to serve them, improve our value proposition, and optimize their experience. Learn more about managing your cookies at Cookies Policy .
1155 Sixteenth Street, NW, Washington, DC 20036, USA | service@acs.org | 1-800-333-9511 (US and Canada) | 614-447-3776 (outside North America)
- Terms of Use
- Accessibility
Copyright © 2024 American Chemical Society
Thank you for visiting nature.com. You are using a browser version with limited support for CSS. To obtain the best experience, we recommend you use a more up to date browser (or turn off compatibility mode in Internet Explorer). In the meantime, to ensure continued support, we are displaying the site without styles and JavaScript.
- View all journals
- Explore content
- About the journal
- Publish with us
- Sign up for alerts
- Research Highlight
- Published: 22 March 2022
ENERGY MATERIALS
Electricity from cells
- Giulia Pacchioni 1
Nature Reviews Materials volume 7 , page 255 ( 2022 ) Cite this article
788 Accesses
8 Altmetric
Metrics details
- Electronic properties and materials
- Materials for energy and catalysis
Photosynthetic cells, if connected to electrodes, can be used to generate electricity. However, designing efficient cell–electrode interfaces is challenging, and much is still not understood about how energy is transferred across them. Now, writing in Nature Materials , Jenny Zhang and collaborators present a 3D-printing method to fabricate hierarchical electrodes that efficiently harness energy from photosynthetic cells.
To investigate the influence of the electrode’s structure, the researchers developed a new versatile fabrication technique to generate large libraries of electrodes. They adapted an aerosol jet printing method — a printing technique for metal nanoparticle inks — to the fabrication of micropillar electrodes made of indium tin oxide (ITO) nanoparticles. ITO is commonly used for bioelectrodes, because it is inert, conducting and biocompatible. The composition and printing parameters of ITO inks were adapted to print 3D structures, and by varying the composition of the ink precursor it was possible to obtain micropillars with different morphologies, ranging from smooth to rough. This technique was used to fabricate arrays of pillars with different heights and degrees of roughness. The electrodes were then incubated with a bacterial culture to attach photosynthetic bacteria on their surface.
This is a preview of subscription content, access via your institution
Access options
Access Nature and 54 other Nature Portfolio journals
Get Nature+, our best-value online-access subscription
24,99 € / 30 days
cancel any time
Subscribe to this journal
Receive 12 digital issues and online access to articles
111,21 € per year
only 9,27 € per issue
Buy this article
- Purchase on Springer Link
- Instant access to full article PDF
Prices may be subject to local taxes which are calculated during checkout
Original article
Chen, X. et al. 3D-printed hierarchical pillar array electrodes for high-performance semi-artificial photosynthesis. Nat. Mater. https://doi.org/10.1038/s41563-022-01205-5 (2022)
Article Google Scholar
Download references
Author information
Authors and affiliations.
Nature Reviews Materials http://www.nature.com/natrevmats/
Giulia Pacchioni
You can also search for this author in PubMed Google Scholar
Corresponding author
Correspondence to Giulia Pacchioni .
Rights and permissions
Reprints and permissions
About this article
Cite this article.
Pacchioni, G. Electricity from cells. Nat Rev Mater 7 , 255 (2022). https://doi.org/10.1038/s41578-022-00436-x
Download citation
Published : 22 March 2022
Issue Date : April 2022
DOI : https://doi.org/10.1038/s41578-022-00436-x
Share this article
Anyone you share the following link with will be able to read this content:
Sorry, a shareable link is not currently available for this article.
Provided by the Springer Nature SharedIt content-sharing initiative
Quick links
- Explore articles by subject
- Guide to authors
- Editorial policies
Sign up for the Nature Briefing newsletter — what matters in science, free to your inbox daily.


- Testing, Performance Tasks, Questions, Webquests
- Discoveries and Inventions - Scientific Phenomena to Use with NGSS
Plants Making Electricity
Scientific Phenomena
Photosynthesis is how plants make food. They take the light from the sun and change it into sugars that they use for energy, growth and repair. They collect the light using special proteins in their chlorophyll . Scientists at MIT have invented a way to make those photosynthetic proteins collect light and instead of making it into sugars, they make it into electricity.
They had to find a way to protect the electrodes from the water and salt that the photosynthetic proteins needed to survive. So they created tiny peptide molecules that would wrap around the photosynthetic proteins like a fish tank keeping them wet and working, while the electrodes stayed dry.
So far, the electrical current they made is weak, but building on this new technology, eventually they hope to make enough power to fuel solar cells for computers and cell phones and well -- anything that can sit out in the sun! (Epstein, David. "Will Your Next Computer Be Powered by Spinach?" Discover January 2005: p. 69)
Off site resource from Discover: http://discovermagazine.com/2005/jan/computer-powered-by-spinach
Now researchers at Technion-Israel Institute of Technology have developed a bio-photo-electro-chemical (BPEC) cell using the a membrane extract from spinach leaves to produce electricity with a hydrogen byproduct. This allows the production of electricity with no combustion and so no carbon dioxide or orther greenhouse gases as a by-product.
(American Technion Society. "Popeye was right: There’s energy in that spinach." ScienceDaily. ScienceDaily, 22 September 2016.)
Off site resource Science Daily: https://www.sciencedaily.com/releases/2016/09/160922085743.htm
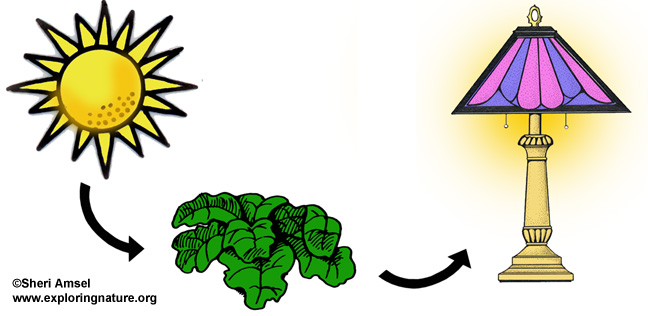
Essential Questions
1. Can we generate electricity from plants?
Disciplinary Core Ideas
PS3.D: Energy in Chemical Processes and Everyday Life : LINK The chemical reaction by which plants produce complex food molecules (sugars) requires an energy input (i.e., from sunlight) to occur. In this reaction, carbon dioxide and water combine to form carbon-based organic molecules and release oxygen. (secondary to MS-LS1-6)
Science and Engineering Practices
Developing and Using Models Modeling in 6–8 builds on K–5 experiences and progresses to developing, using, and revising models to describe, test, and predict more abstract phenomena and design systems. • Develop and use a model to describe phenomena. (MS-LS1-2) • Develop a model to describe unobservable mechanisms. (MS-LS1-7) Planning and Carrying Out Investigations Planning and carrying out investigations in 6-8 builds on K-5 experiences and progresses to include investigations that use multiple variables and provide evidence to support explanations or solutions. • Conduct an investigation to produce data to serve as the basis for evidence that meet the goals of an investigation. (MS-LS1-1)
Constructing Explanations and Designing Solutions : Apply scientific ideas to construct an explanation for real-world phenomena, examples, or events. Obtaining, Evaluating, and Communicating Information : Gather, read, and synthesize information from multiple appropriate sources and assess the credibility, accuracy, and possible bias of each publication and methods used, and describe how they are supported or not supported by evidence.
Crosscutting Concepts
Cause and Effect : Phenomena may have more than one cause, and some cause and effect relationships in systems can only be described using probability.
Systems and System Models • Systems may interact with other systems; they may have sub-systems and be a part of larger complex systems. (MS-LS1-3) Energy and Matter • Matter is conserved because atoms are conserved in physical and chemical processes. (MS-LS1-7) • Within a natural system, the transfer of energy drives the motion and/or cycling of matter. (MS-LS1-6) Structure and Function • Complex and microscopic structures and systems can be visualized, modeled, and used to describe how their function depends on the relationships among its parts, therefore complex natural structures/systems can be analyzed to determine how they function. (MS-LS1-2)
Other questions that were generated in researching this Scientific Phenomena?
High Resolution PDF for Printing
Intergrate this lesson with ngss:.
______________________________________________________________________________
Using Discoveries and Inventions as Scientific Phenomena to Integrate with NGSS: ______________________________________________________________________________ Scientific Phenomena can be used as a tool to anchor a science unit involving a series of lessons to engage in deeper science learning – or what is being called “Three Dimensional Learning”. 1) Describe the phenomena in a way that your students can understand and which sparks their imagination. 2) Create Essential Questions for them to answer to explain the phenomena. 3) Identify the NGSS Disciplinary Core Ideas which you are targeting. 4) Provide clear directions for a process they should use to try to answer the questions using the NGSS Science and Engineering Practices to frame as your guideline.
5) Name the Crosscutting Concepts that students should be aware of throughout the lesson.
6) Discuss the Connections to Nature of Science. 7) Make note of other questions generated in the process of exploring this Scientific Phenomena .
Citing Research References
When you research information you must cite the reference. Citing for websites is different from citing from books, magazines and periodicals. The style of citing shown here is from the MLA Style Citations (Modern Language Association).
When citing a WEBSITE the general format is as follows. Author Last Name, First Name(s). "Title: Subtitle of Part of Web Page, if appropriate." Title: Subtitle: Section of Page if appropriate. Sponsoring/Publishing Agency, If Given. Additional significant descriptive information. Date of Electronic Publication or other Date, such as Last Updated. Day Month Year of access < URL >.
Here is an example of citing this page:
Amsel, Sheri. "Plants Making Electricity" Exploring Nature Educational Resource ©2005-2024. April 23, 2024 < http://www.exploringnature.org/db/view/Plants-Making-Electricity >
Exploringnature.org has more than 2,000 illustrated animals. Read about them, color them, label them, learn to draw them.

How to generate electricity from the roots of living plants
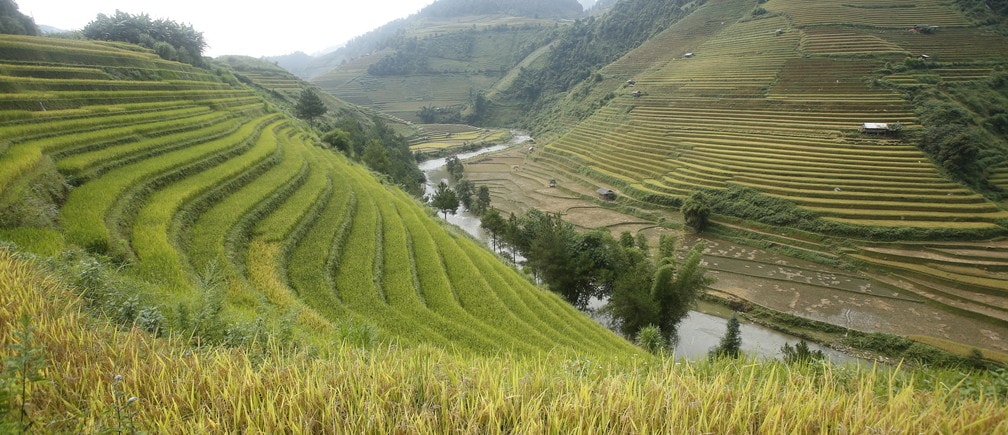
"No more competing claims for food versus fuel: put both rice production and power on the same paddy." Image: REUTERS/Kamal Kishore
.chakra .wef-1c7l3mo{-webkit-transition:all 0.15s ease-out;transition:all 0.15s ease-out;cursor:pointer;-webkit-text-decoration:none;text-decoration:none;outline:none;color:inherit;}.chakra .wef-1c7l3mo:hover,.chakra .wef-1c7l3mo[data-hover]{-webkit-text-decoration:underline;text-decoration:underline;}.chakra .wef-1c7l3mo:focus,.chakra .wef-1c7l3mo[data-focus]{box-shadow:0 0 0 3px rgba(168,203,251,0.5);} Marjolein Helder
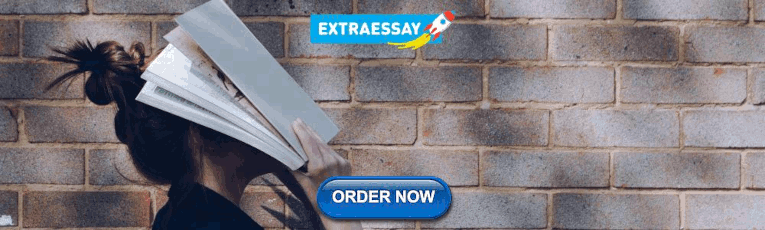
.chakra .wef-9dduvl{margin-top:16px;margin-bottom:16px;line-height:1.388;font-size:1.25rem;}@media screen and (min-width:56.5rem){.chakra .wef-9dduvl{font-size:1.125rem;}} Explore and monitor how .chakra .wef-15eoq1r{margin-top:16px;margin-bottom:16px;line-height:1.388;font-size:1.25rem;color:#F7DB5E;}@media screen and (min-width:56.5rem){.chakra .wef-15eoq1r{font-size:1.125rem;}} Future of the Environment is affecting economies, industries and global issues
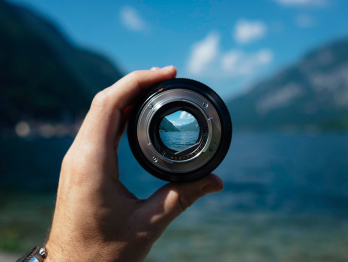
.chakra .wef-1nk5u5d{margin-top:16px;margin-bottom:16px;line-height:1.388;color:#2846F8;font-size:1.25rem;}@media screen and (min-width:56.5rem){.chakra .wef-1nk5u5d{font-size:1.125rem;}} Get involved with our crowdsourced digital platform to deliver impact at scale
Stay up to date:, future of the environment.
Somewhere between three and four billion years ago, algae first appeared. This may not sound exciting, but it paved the way for life on earth and could ultimately point to part of the solution to today’s energy crisis.
Algae, along with other early organisms like cyaonobacteria, is photosynthetic. This means that it’s able to capture carbon and breath out oxygen in return. And we need oxygen to breathe. During the following billions of years, more photosynthetic organisms developed: plants.
The interesting thing about plants is that they convert CO2 into chemical energy - glucose - and produce water and oxygen. So basically plants do what we’re struggling to achieve, in a world threatened by climate change: they capture CO2, produce energy and keep our air clean and breathable. So why can’t we do the same thing? Well, we can’t “do” photosynthesis, but we can use it.
How it all started
At Wageningen University in the Netherlands, a crazy assistant professor, Bert Hamelers, thought that it should be possible to produce electricity from living plants, without harvesting them. He hired a Postdoc to do an experiment and he succeeded. They wrote a research proposal and hired a PhD-student to do more work on it: me.
I didn’t want to be in academia (who wants to be in a lab all day?) but not knowing what to do otherwise I ended up doing a PhD. Basically, my professor lured me into it by telling me that a spin-off company should be created from the research project, and he thought I would be fit to lead it. After just one month, I knew that I’d made the best decision of my life.
How it works
So how do you produce electricity with living plants? Simply by using the natural processes that already occur. In short: the plant produces organic matter via photosynthesis. Only part of this organic matter is then used for its own growth. The rest is excreted via the roots. Around the roots, bacteria feed on the organic matter and they release electrons. If you’re able to harvest the electrons into an electrode, you can couple the first electrode to a counter-electrode and build an electrical circuit, like in a battery. The electrons flow back into the natural system via the counter-electrode, so it’s completely circular.
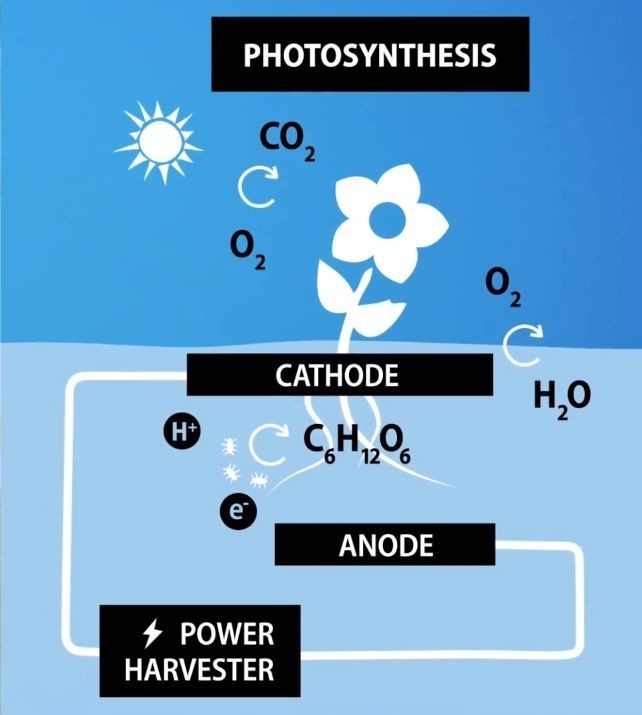
Because we use the natural processes around the plant, nature is not harmed. It works day and night, summer and winter. It only stops when the plant and its surroundings completely dry up or freeze over. So wetlands would be the ultimate source of electricity. Probably the best thing about this technology is that it can be combined with existing applications for the same land. No more competing claims for food versus fuel: put both rice production and power on the same paddy.
How we can use it
During my PhD, I worked on improving the power output from plants in the lab. At the same time I started a spin-off company, Plant-e, together with my colleague David Strik, to find applications for the technology. When I graduated in 2012 we launched the first product: a turning globe fuelled by the electricity from a plant. Unfortunately, at that time we couldn’t get the product produced so our first market entry failed. But we were able to attract some financing, so we hired some smart young people and worked on the next product.
In 2014 the first product was launched successfully: a modular system. Basically we sell planters with plants and wires that can be connected to LED lights, for example. This is not going to replace coal-fired power plants, but it’s a start. This is the first step towards using what nature has developed over billions of years, without interfering with nature.
The modular system can be used to set up small, self-powered sites in cities, but it is not scalable. So a new system is under development. This new system is a tube, which contains both electrodes and can be drilled horizontally in the root-zone of the plants. This way existing plants can be used to produce electricity and any wetland would ultimately be able to produce electricity.
The next energy revolution?
After wind, solar and hydropower, the full range of biomass sources are now ready for energy production. Our energy revolution has already started. We go from large scale to decentralized energy production, and we start to realize that no one individual source is going to save us.
It’s going to be the full range of alternative, renewable and sustainable technologies that are slowly replacing fossil fuels. I don’t think that one small company of five people in Wageningen, the Netherlands, is going to revolutionize our energy production. But I do want to be part of it.
Author: Marjolein Helder is the CEO of Plant-e, a World Economic Forum Technology Pioneer. She is participating in the World Economic Forum’s Annual Meeting in Davos.
Don't miss any update on this topic
Create a free account and access your personalized content collection with our latest publications and analyses.
License and Republishing
World Economic Forum articles may be republished in accordance with the Creative Commons Attribution-NonCommercial-NoDerivatives 4.0 International Public License, and in accordance with our Terms of Use.
The views expressed in this article are those of the author alone and not the World Economic Forum.
Related topics:
The agenda .chakra .wef-n7bacu{margin-top:16px;margin-bottom:16px;line-height:1.388;font-weight:400;} weekly.
A weekly update of the most important issues driving the global agenda
.chakra .wef-1dtnjt5{display:-webkit-box;display:-webkit-flex;display:-ms-flexbox;display:flex;-webkit-align-items:center;-webkit-box-align:center;-ms-flex-align:center;align-items:center;-webkit-flex-wrap:wrap;-ms-flex-wrap:wrap;flex-wrap:wrap;} More on Nature and Biodiversity .chakra .wef-17xejub{-webkit-flex:1;-ms-flex:1;flex:1;justify-self:stretch;-webkit-align-self:stretch;-ms-flex-item-align:stretch;align-self:stretch;} .chakra .wef-nr1rr4{display:-webkit-inline-box;display:-webkit-inline-flex;display:-ms-inline-flexbox;display:inline-flex;white-space:normal;vertical-align:middle;text-transform:uppercase;font-size:0.75rem;border-radius:0.25rem;font-weight:700;-webkit-align-items:center;-webkit-box-align:center;-ms-flex-align:center;align-items:center;line-height:1.2;-webkit-letter-spacing:1.25px;-moz-letter-spacing:1.25px;-ms-letter-spacing:1.25px;letter-spacing:1.25px;background:none;padding:0px;color:#B3B3B3;-webkit-box-decoration-break:clone;box-decoration-break:clone;-webkit-box-decoration-break:clone;}@media screen and (min-width:37.5rem){.chakra .wef-nr1rr4{font-size:0.875rem;}}@media screen and (min-width:56.5rem){.chakra .wef-nr1rr4{font-size:1rem;}} See all
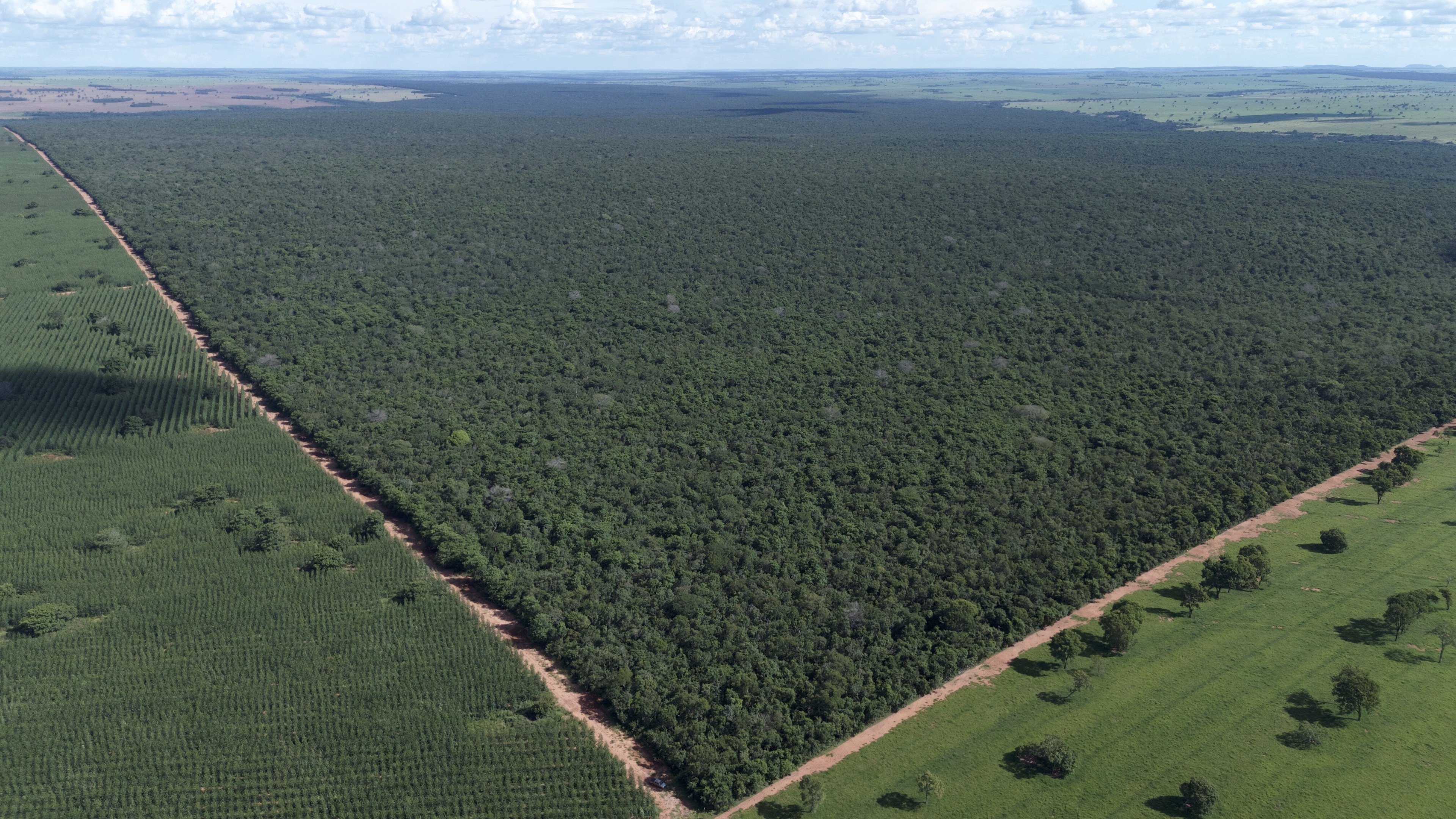
5 ways sustainable forestry can support climate action, development and biodiversity
Charlotte Kaiser
April 23, 2024
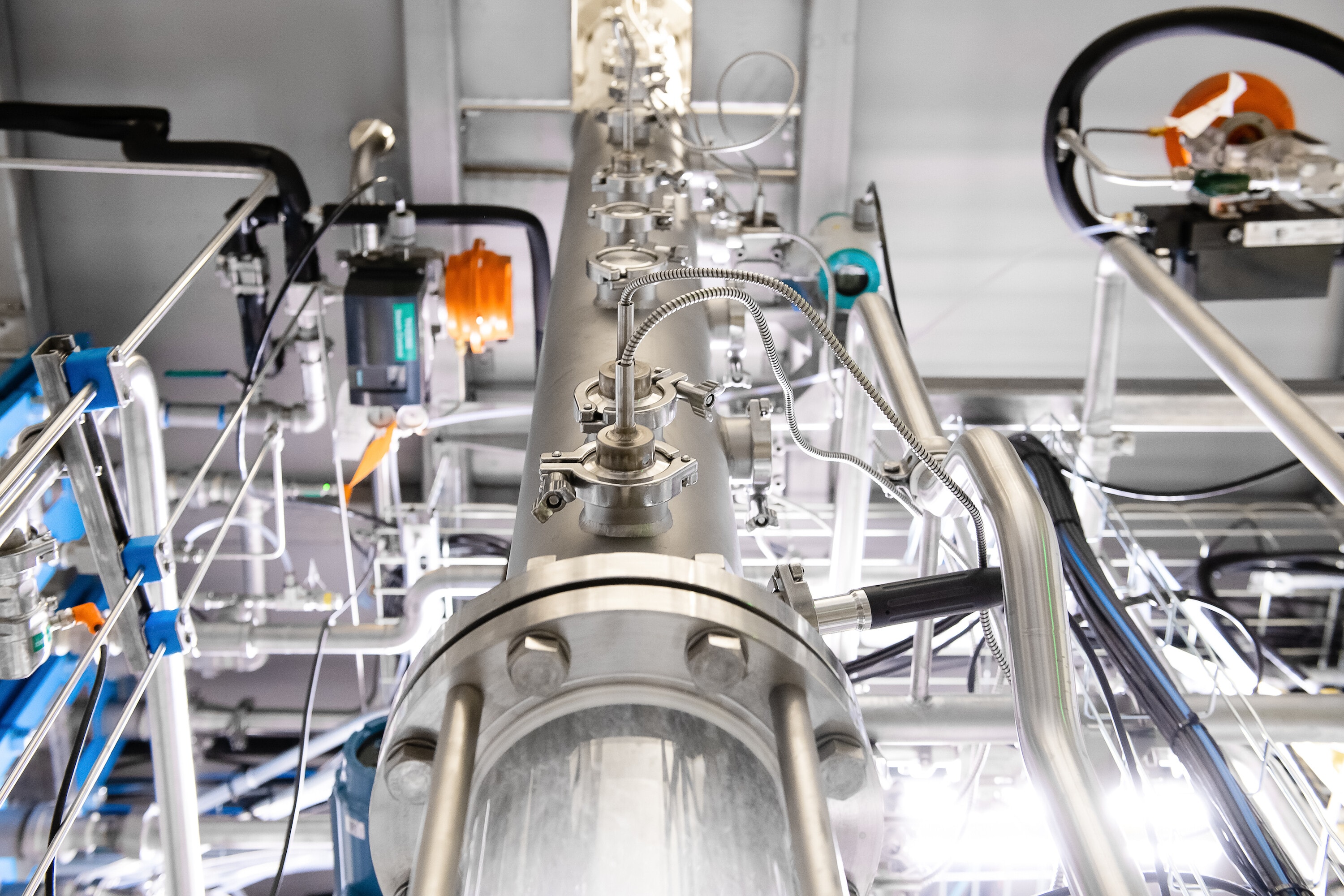
4 lessons from the renewables playbook for today’s clean infrastructure boom
Jennifer Holmgren
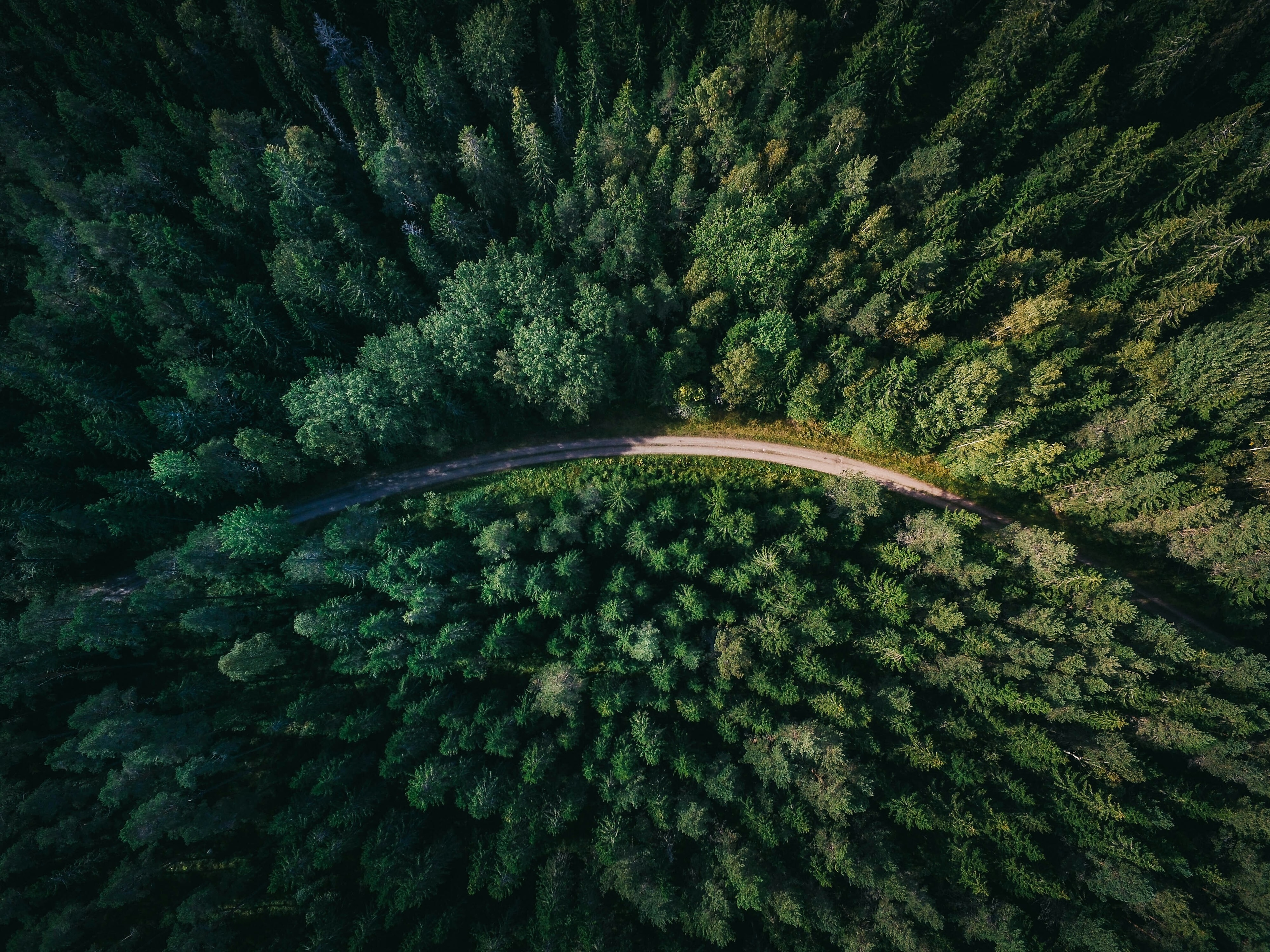
Global forest restoration goals can be achieved with youth-led ecopreneurship
Agustin Rosello, Anali Bustos, Fernando Morales de Rueda, Jennifer Hong and Paula Sarigumba
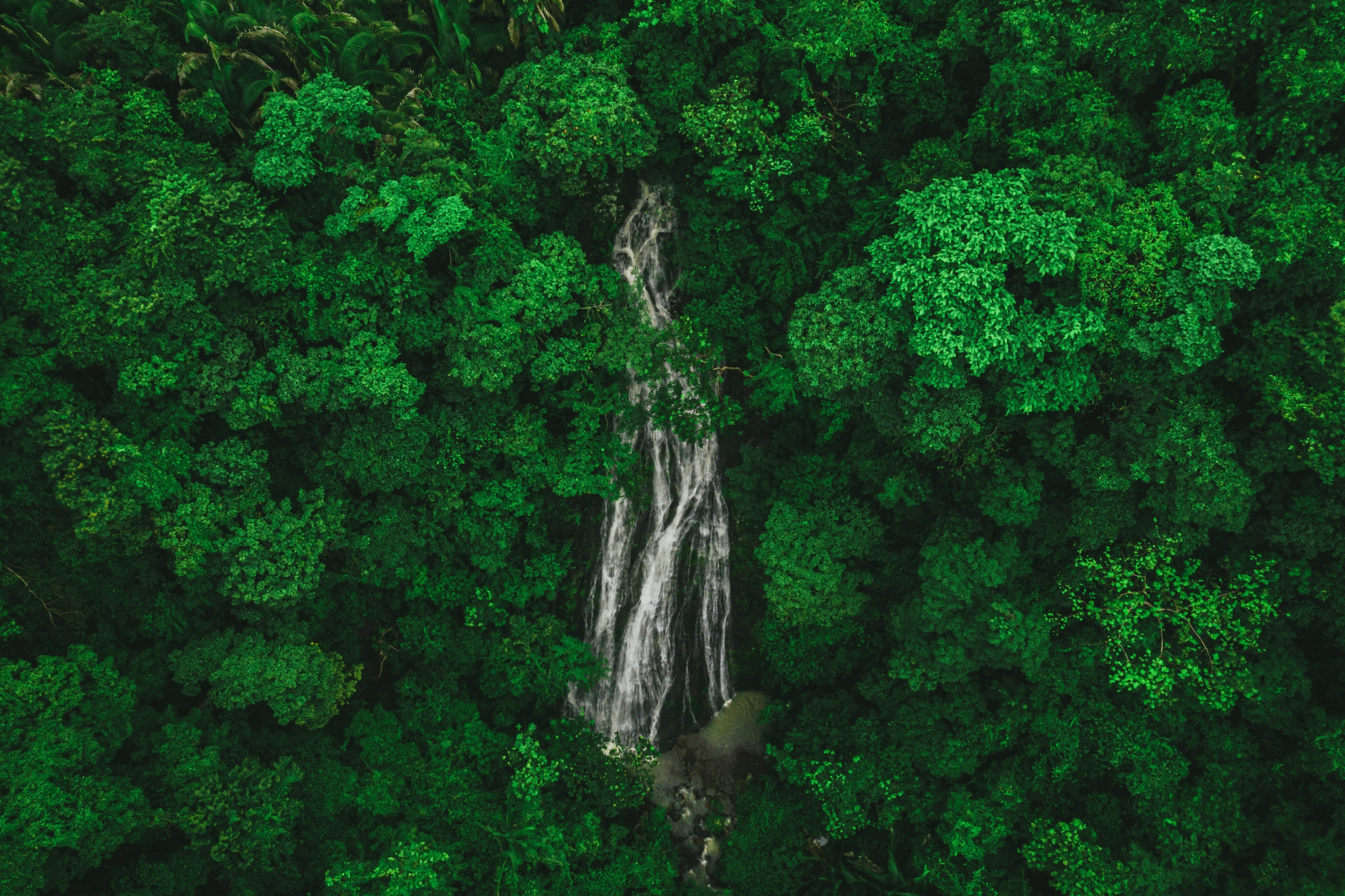
The planet’s outlook is in our hands. Which future will we incentivize?
Carlos Correa
April 22, 2024
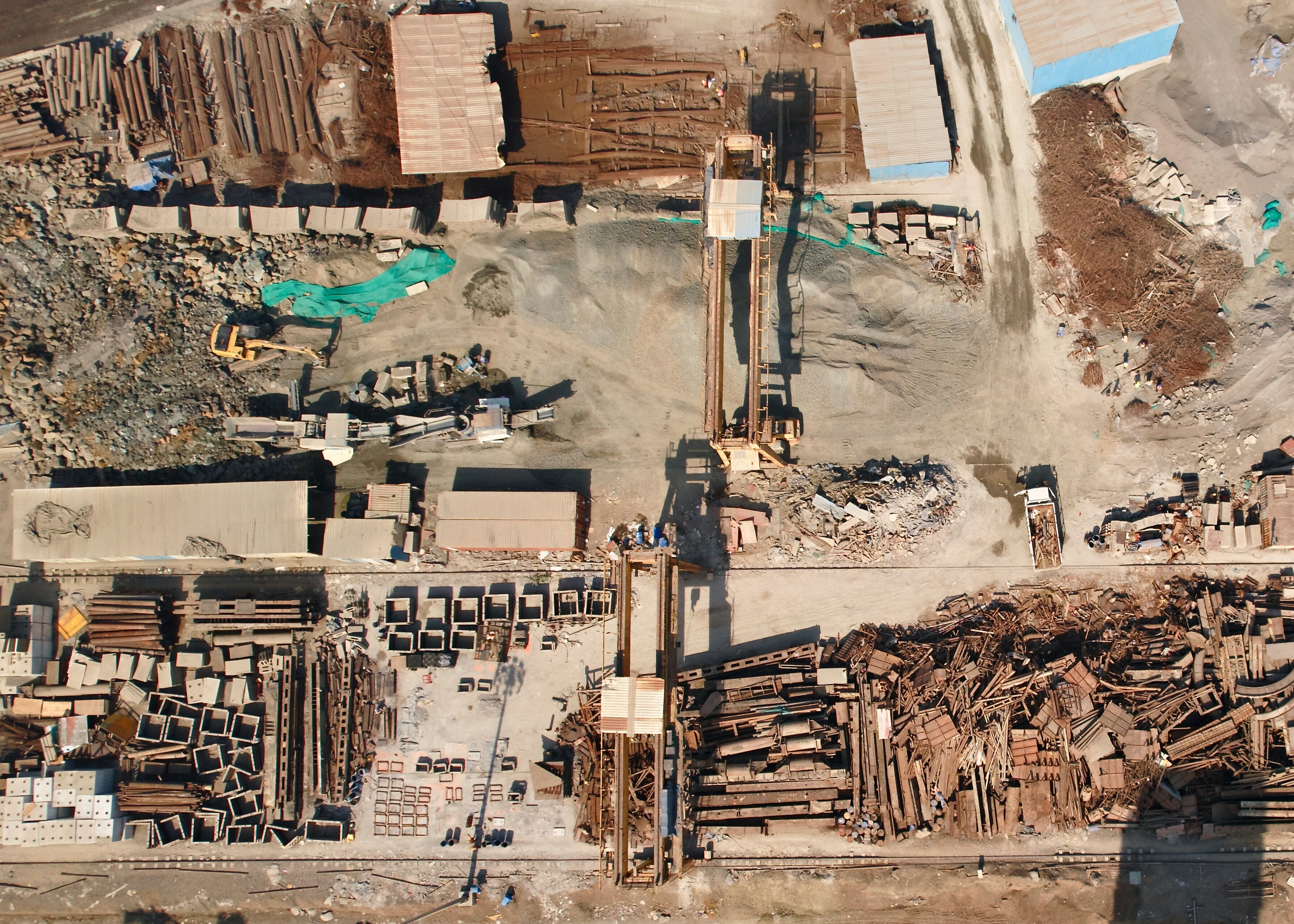
Why recycling metal is an opportunity too good to waste
Nick Pickens and Julian Kettle
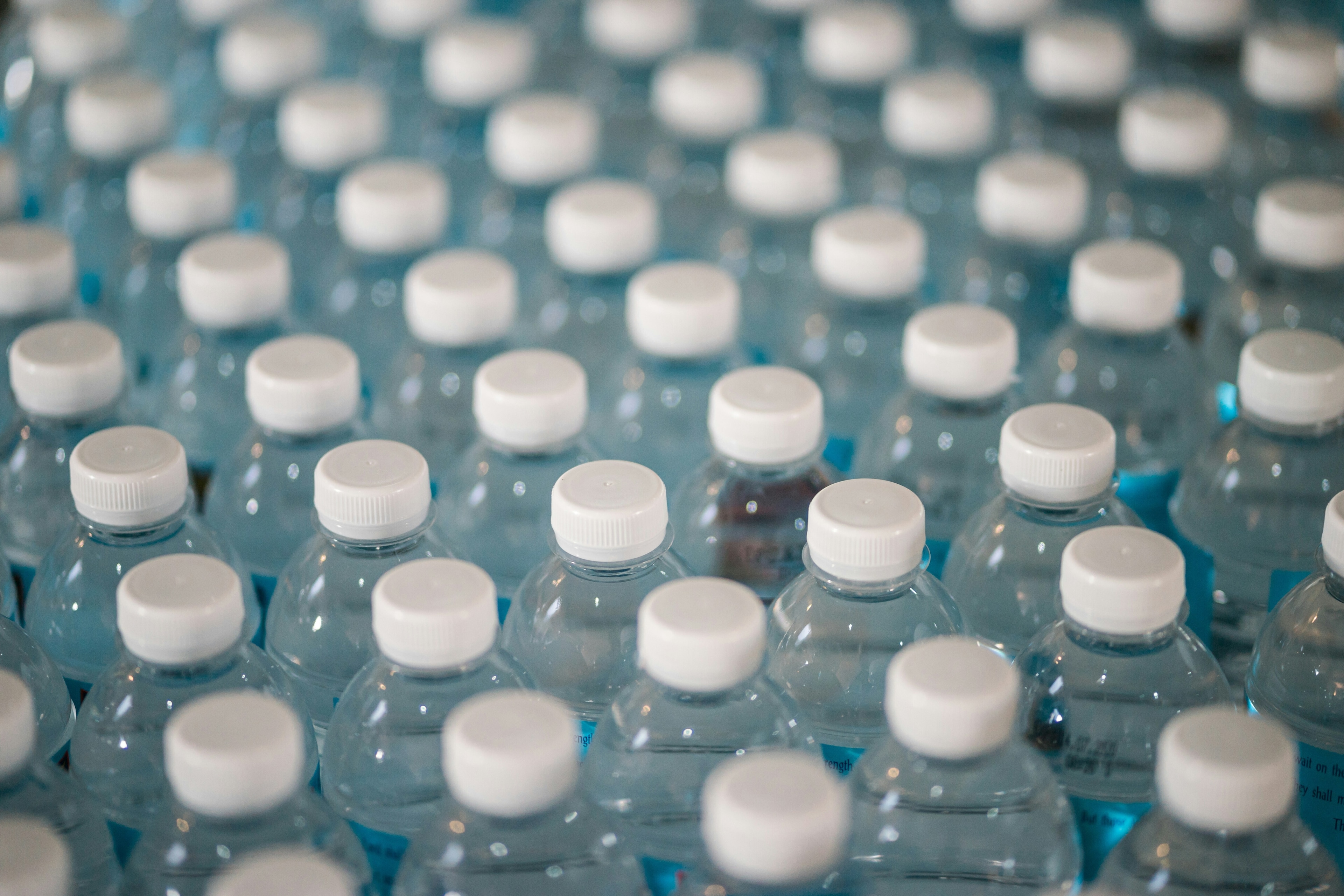
Earth Day 2024: These 4 innovators have big ideas for developing a sustainable plastics ecosystem
Simon Torkington
Advertisement
Electrochemistry of plants: basic theoretical research and applications in plant science
- Review Paper
- Published: 13 September 2021
- Volume 25 , pages 2747–2757, ( 2021 )
Cite this article
- Antonio Doménech-Carbó ORCID: orcid.org/0000-0002-5284-2811 1
596 Accesses
5 Citations
Explore all metrics
Electrochemistry of plants can be viewed as a multifaceted research fields including electrophysiological studies around signaling in plants and those associated with the interaction of plants with the environment. Apart from botanic, this research can be implemented into agriculture, food chemistry, ecology, climatology, and other fields with a variety of applications. Vegetal matter can also be applied in sensors, transducers, and as a source for preparing materials to be applied in sensing, energy production, and storage. These aspects are shortly reviewed, including those specifically dealing with solid-state electrochemistry and their capabilities in regard to chemoecological and phylogenetic issues.
This is a preview of subscription content, log in via an institution to check access.
Access this article
Price includes VAT (Russian Federation)
Instant access to the full article PDF.
Rent this article via DeepDyve
Institutional subscriptions
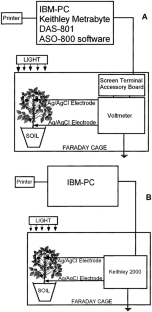
Similar content being viewed by others
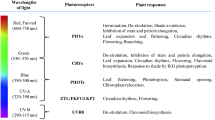
Light-Quality Manipulation to Control Plant Growth and Photomorphogenesis in Greenhouse Horticulture: The State of the Art and the Opportunities of Modern LED Systems
Roberta Paradiso & Simona Proietti
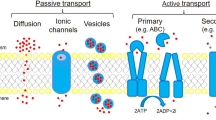
Root exudates: from plant to rhizosphere and beyond
Vicente Vives-Peris, Carlos de Ollas, … Rosa María Pérez-Clemente
Plant Growth Regulators: Backgrounds and Uses in Plant Production
Wilhelm Rademacher
Volkov AG (ed) (2006) Plant electrophysiology theory and methods. Springer, Berlin-Heidelberg
Google Scholar
Amatore C, Arbault S, Guille M, Lemaître F (2008) Electrochemical monitoring of single cell secretion: vesicular exocytosis and oxidative stress. Chem Rev 108:2585–2621
Article CAS PubMed Google Scholar
Scholz F, Meyer B (1998) Voltammetry of solid microparticles immobilized on electrode surfaces, Electroanalytical Chemistry, A Series of Advances. In: AJ Bard, I Rubinstein (eds) Marcel Dekker, New York 20:1−86
Scholz F, Schröder U, Gulaboski R, Doménech-Carbó A (2015) Electrochemistry of immobilized particles and droplets, 2nd edn. Springer International Publishing, Berlin-Heidelberg
Book Google Scholar
Doménech-Carbó A, Labuda J, Scholz F (2013) Electroanalytical chemistry for the analysis of solids: characterization and classification (IUPAC Technical Report). Pure Appl Chem 85:609–631
Article Google Scholar
Komorsky-Lovrić Š, Novak I (2009) Estimation of antioxidative properties of tea leaves by abrasive stripping electrochemistry using paraffin-impregnated graphite electrode. Collect Czech Chem Commun 74:1467–1475
Komorsky-Lovrić Š, Novak I (2011) Abrasive stripping square-wave voltammetry of blackberry, raspberry, strawberry, pomegranate, and sweet and blue potatoes. J Food Sci 76:C916–C920
Article PubMed Google Scholar
Doménech-Carbó A, Domínguez I, Hernández P, Gavara R (2015) Electrochemical tomato ( Solanum lycopersicum L.) characterization using contact probe in situ voltammetry. Food Chem 127:318–325
Domínguez I, Doménech-Carbó A (2015) Screening and authentication of tea varieties based on microextraction-assisted voltammetry of microparticles. Sensors Actuator B 210:491–499
Milczarek G (2009) Preparation, characterization and electrocatalytic properties of an iodine|lignin-modified gold electrode. Electrochim Acta 54:3199–3205
Article CAS Google Scholar
Cottyn B, Rivard M, Majira A, Beauhaire J, Allais F, Martens T, Baumberger S, Ducrot PH (2015) Comparative electrochemical study on monolignols and rticul relevant for the comprehension of the lignification. Phytochem Lett 13:280–285
Kilmartin PA, Hsu CF (2003) Characterisation of polyphenols in green, oolong, and black teas, and in coffee, using cyclic voltammetry. Food Chem 82:501–512
Grygar T, Kucková S, Hradil D, Hradilová D (2003) Electrochemical analysis of natural solid organic dyes and pigments. J Solid State Electrochem 7:706–713
Doménech-carbó A, Doménech-Carbó MT, Saurí-Peris MC (2005) Electrochemical identification of flavonoid dyes in work of art samples by abrasive voltammetry at paraffin-impregnated graphite electrodes. Talanta 66:769–782
Stahlberg R (2006) Historical introduction to plant electrophysiology, In Volkov AG (ed) (2006) Plant electrophysiology theory and methods. Springer, Berlin-Heidelberg
Bois-Reymond Du (1848) Untersuchungen rti thierische Elektrizitat. Reimer, Berlin
Pfeffer W (1873) Physiologische Untersuchungen. Engelmann, Leipzig
Nernst W (1889) Die elektromotorische Wirksamkeit der Ionen. Z Phys Chem 4:129–181
Bernstein J (1912) Elektrobiologie. Thieme, Braunschweig
Umrath K (1930) Untersuchungen rti Plasma und Plasamstromung a rticulat. IV. Potentialmessungen an Nitella mucronata mit besonderer Berucksichtigung der Erregungserscheinungen. Protoplasma 9:576–597
Osterhout WJV (1936) Electrical phenomena in large plant cells. Physiol Rev 16:216–237
Montenegro MI, Queiros MA, Daschbach JL (1991) Microelectrodes: theory and applications. Kluwer Articula, Dordrecht
Higinbotham N (1973) Electropotentials of plant cells. Annu Rev Plant Physiol 24:25–46
Urticu AJ, Cookson SJ, Smith SJ, Wells DM (2001) The use of microelectrodes to investigate compartmentation and the transport of articulate inorganic ions in plants. J Exp Bot 52:541–549
Miller AJ, Wells DM (2006) Electrochemical methods and measuring transmembrane ion gradients. In: Volkov AG (ed) (2006) Plant electrophysiology theory and methods. Springer, Berlin-Heidelberg
Coster HGL (1966) Chloride in cells of Chara australis . Aust J Biol Sci 19:545–554
Kumar SM, Porterfield DM, Muller KJ, Smith PJ, Sahley CL (2001) Nerve injury induces a rapid efflux of nitric oxide (NO) detected with a novel NO microsensor. J Neurosci 21:215–220
Article CAS PubMed PubMed Central Google Scholar
Orticu S (2006) Non-invasive microelectrode ion flux measurements in plant stress physiology. In: Volkov AG (ed) (2006) Plant electrophysiology theory and methods. Springer, Berlin-Heidelberg
Lucas WJ, Kochian LV (1986) Ion transport processes in corn roots: an approach utilizing microelectrode techniques. In: Gensler WG (ed) Advanced agricultural instrumentation: design and use. Nijhoff, Dordrecht, pp 402–425
Chapter Google Scholar
Newman IA, Kochian LV, Grusak MA, Lucas WJ (1987) Fluxes of H + and K + in corn roots. Characterisation and stoichiometries using ion-selective microelectrodes. Plant Physiol 84:1177–1184
Lindner E, Gyurcsanyi RE, Buck RP (1999) Tailored transport through ion-selective membranes for improved detection limits and selectivity coefficients. Electroanalysis 11:695–702
Land SC, Porterfield DM, Sanger RH, Smith PJS (1999) The self-referencing oxygen-selective microelectrode: detection of transmembrane oxygen flux from single cells. J Exp Biol 202:211–218
Jung S-K, Hammar K, Smith PJS (2000) Development of self-referencing oxygen microsensor and its application to single HIT cells. Biol Bull 199:197–198
Demidchik V, Sokolik A, Yurin V (2006) electrophysiological characterization of plant cation channels, In Volkov AG, Ed (2006) Plant electrophysiology theory and methods. Springer, Berlin-Heidelberg
Mancuso S, Marras AM (2006) New solid state microsensors in plant physiology. In: Volkov AG (ed) (2006) Plant electrophysiology theory and methods. Springer, Berlin-Heidelberg
Papeschi G, Mancuso S, Marras AM (2000) Electrochemical behaviour of Cu/CuSe microelectrode and its application in detecting temporal and spatial articulate of copper(II) fluxes along Olea europaea roots. J Solid State Electrochem 4:325–329
Dutta D, Landolt D (1972) Electrochemical behavior of nitric oxide in 4 M H2SO4 on platinum. J Electrochem Soc 119:1320–1325
Zhang XL, Cardoso L, Broderick M, Fein F, Lin J (2000) Novel integrated nitric oxide sensor based on carbon fiber electrode coated with NO-selective membranes. Electroanalysis 12:1113–1117
Mancuso S, Marras AM, Volker M, Baluska F (2005) Non-invasive and continuous recordings of auxin fluxes in intact root apex with a carbon-nanotube-modified and self-referencing microelectrode. Anal Biochem 341:344–351
Lima AS, Prieto KR, Santos CS, Valerio HP, Garcia-Ochoa EY, Huerta-Robles A, Beltran-Garcia MJ, Di Mascio P, Bertotti M (2018) In-vivo electrochemical monitoring of H 2 O 2 production induced by root-inoculated endophytic bacteria in Agave tequilana leaves. Biosens Bioelectr 99:108–114
Liu X, Liu X, Wei H, Song G, Guo H, Lu X (2017) Sensitive detection of superoxide anion released from living cells using silver nanoparticles and functionalized multiwalled carbon nanotube composite. Sensors Actuator B 252:503–510
Volkov AG, Volkova-Gugeshashvili MI, Brown-McGauley CN, Osei AJ (2007) Nanodevices in nature: electrochemical aspects. Electrochim Acta 52:2905–2912
Volkov AG (2000) Green plants: electrochemical interfaces. J Electroanal Chem 483:150–156
Volkov AG, Dunkley TC, Labady AJ, Brown CL (2005) Phototropism and electrified interfaces in green plants. Electrochim Acta 50:4241–4247
Copedè N, Janni M, Bettelli M, Maida CL, Gentile F, Villani M, Ruotolo R, Iannotta S, Marmiroli N, Marmiroli M, Zappettini A (2017) An in vivo biosensing, biomimetic electrochemical transistor with applications in plant science and precision farming. Sci Rep 7(16195)
Yasukawa T, Kaya T, Matsue T (2000) Characterization and imaging of single cells with scanning electrochemical microscopy. Electroanalysis 12:653–659
Tsionsky M, Cardon ZG, Bard AJ, Jackson RB (1997) Photosynthetic electron transport in single guard cells as measured by scanning electrochemical microscopy. Plant Physiol 113:895–901
Zhu R, Macfie SM, Ding Z (2005) Cadmium-induced plant stress investigated by scanning electrochemical microscopy. J Exp Bot 56:2831–2838
Wilson GS, Johnson MA (2008) In-vivo electrochemistry: what can we learn about living systems? Chem Rev 108:2462–2481
Zhang W, Xu B, Hong Y, Yu Y, Ye J, Zhang J (2010) Electrochemical determination of salicylic acid at a new biosensor based on polypyrrole-banana tissue composite. J Solid State Electrochem 14:1713–1718
Lu S, Bai L, Wen Y, Li M, Yan D, Zhang R, Chen K (2015) Water-dispersed carboxymethyl cellulose-montmorillonite-single walled carbon nanotube composite with enhanced sensing performance for simultaneous determination of two trace phytohormones. J Solid State Electrochem 19:2023–2037
Alizadeh T, Nayeri S (2018) Electrocatalytic oxidation of salicylic acid at a carbon paste electrode impregnated with cerium-doped zirconium oxide nanoparticles as a new sensing approach for salicylic acid determination. J Solid State Electrochem 22:2039–2048
Liao Y-Q, Wang F, Chen Z-L (2010) Electrochemical behavior and the determination of methyl jasmonate. Chin Sci Bull 55:2225–2230
Ballaré CL (2011) Jasmonate-induced defenses: a tale of intelligence, collaborators and rascals. Trends Plant Sci 16:249–257
Koornneef A, Leon-Reyes A, Ritsema T, Verhage A, Den Otter FC, Van Loon LC, Pieterse CMJ (2008) Kinetics of salicylate-mediated supression of jasmonate signaling reveal a role for redox modulation. Plant Physiol 147:1358–1368
Kawazu K, Mochizuki A, Sato Y, Sugeno W, Murata M, Seo S, Mitsuhara I (2012) Different articulate profiles of jasmonic acid and salicylic acid inducible genes in the tomato plant against herbivores with various feeding modes. Arthropod-Plant Inte 6:221–230
Doménech-Carbó A, Dias D, Donnici M (2021) In vivo electrochemical monitoring of signaling transduction of plant defense against stress in leaves of Aloe vera L. Electroanalysis 33:1024–1032
Yang B, Kotani A, Arai K, Kusu F (2001) Estimation of the antioxidant activities of flavonoids from their oxidation potentials. Anal Sci 17:599–604
Brainina KhZ, Ivanova AV, Sharafutdinova EN, Lozovskaya EL, Shkarina EI (2007) Potentiometry as a method of antioxidant activity investigation. Talanta 71:13–18
Glod BK, Kiersztyn I, Piszcz P (2014) Total antioxidant potential assay with cyclic voltammetry and/or differential pulse voltammetry measurements. J Electroanal Chem 719:24–29
Korotkova EI, Voronova OA, Dorozhko EV (2012) Study of antioxidant properties of flavonoids by voltammetry. J Solid State Electrochem 16:2435–2440
Blasco AJ, Rogelio MC, González MC, Escarpa A (2005) “Electrochemical Index” as a screening method to determine “total polyphenolics” in foods: a proposal. Anal Chim Acta 539:237–244
Wang Y, Hasebe Y (2011) Acridine orange-induced signal enhancement effect of tyrosinase-immobilized carbon-felt-based flow biosensor for highly sensitive detection of monophenolic compounds. Anal Bioanal Chem 399:1151–1162
Villela Maciel J, Almeida Silva T, Dias D, Fatibello-Filho O (2018) Electroanalytical determination of eugenol in clove oil by voltammetry of immobilized microdroplets. J Solid State Electrochem 22:2277–2285
Scholz F, González GLL, de Carvalho LM, Hilgemann M, Brainina KhZ, Kahlert H, Jack RS, Minh DT (2007) An electrochemical system to detect free radicals and radical scavengers in solution. Angew Chem Int Ed 46:8079–8082
de Carvalho LM, Hilgemann M, Scholz F, Kahlert H, da Rosa MB, Wuster M, Lindequist U, do Nascimento PC, Bohrer D, (2010) Electrochemical assay to quantify the hydroxyl radical scavenging activity of medicinal plant extracts. Electroanalysis 22:406–412
Sanchez LM, Doke N, Kawakita K (1993) Elicitor-induced chemiluminescence in cell horticulate cultures of tomato, sweet pepper and tobacco plants and its inhibition by suppressors from Phytophthora spp. Plant Sci 88:141–148
Otte O, Barz W (1996) The elicitor-induced oxidative burst in cultured chickpea cells dricves the rapid insolubilization of two cell wall structural proteins. Planta 200:238–246
Nakamura N, Kumazawa S, Sode K, Matsunaga T (1993) Development of rtic detection system for allergic reaction using rat basophilic rticula (RBL-1) cells. Sensors Actuator B 13–14:312–314
Matsue T, Matsumoto N, Koike S, Uchida I (1993) Micro-ring electrode for manipulation of a single cell. Biochim Biophys Acta 1157:332–335
Kinpara T, Murakami Y, Yokoyama K, Tamiya E (2000) Electrochemical Monitoring of Plant Stress Responses. Electroanalysis 13:451–456
Marsellis B, Garcia-Gomez J, Michaud PA, Rodrigo MA, Comninellis C (2003) Electrogeneration of hydroxyl radicals on boron-doped diamond electrodes. J Electrochem Soc 150:D79–D83
Enache TA, Chiorcea-Paquim AM, Fatibello-Filho O, Oliveira-Brett AM (2009) Hydroxyl radicals electrochemically generated in situ on a boron doped diamond electrode. Electrochem Commun 11:1342–1345
Kapałka A, Foti G, Comninellis C (2009) The importance of electrode material in environmental electrochemistry: Formation and reactivity of free hydroxyl radicals on boron-doped diamond electrodes. Electrochim Acta 54:2018–2023
Le Bourvellec C, Hauchard D, Darchen A, Burgot J-L, Abasq M-L (2008) Validation of a new method using the reactivity of electrogenerated superoxide radical in the antioxidant capacity determination of flavonoids. Talanta 75:1098–1110
Doménech-Carbó A, Gavara R, Hernández P, Domínguez I (2015) Contact probe voltammetry for in situ monitoring of the reactivity of phenolic tomato ( Solanum lycopersicum L.) compounds with ROS. Talanta 144:1207–1215
Doménech-Carbó A, Cervelló P, González JM, Soriano P, Estrelles E, Montoya N (2019) Electrochemical monitoring of ROS influence on seedlings and germination response to salinity stress of three species of the tribe Inuleae. RSC Adv 9:17856–17867
Maciel JV, Fava EL, Silva TA, Dias D, Fatibello-Filho O (2017) A combination of voltammetry of immobilized microparticles and carbon black-based crosslinked chitosan films deposited on glassy carbon electrode for the quantification of hydroquinone in dermatologic cream samples. J Solid State Electrochem 21:2859–2868
Perumal P, Christopher Selvin P (2020) Red algae-derived k-carrageenan-based protonconducting electrolytes for the wearable electrical devices. J Solid State Electrochem 24:2249–2260
Kiruthika S, Malathi M, Selvasekarapandian S, Tamilarasan K, Moniha V, Manjuladevi R (2019) Eco-friendly biopolymer electrolyte, pectin with magnesium nitrate salt, for application in electrochemical devices. J Solid State Electrochem 23:2181–2193
Siqueira do Nascimento Marreiro Teixeira AS, Sousa Teixeira PR, de Oiveira Farias EA, Ferraz e Sousa B, Bonfim de Leite Moura Sérvulo K, Alves da Silva D, Eiras C (2020) Babassu mesocarp (Orbignya phalerata Mart) nanoparticle-based biosensors for indirect sulfite detection in industrial rticu. J Solid State Electrochem 24:1143–1155
Vieira Jodar L, Santos FA, Zucolotto V, Campos Janegitz B (2018) Electrochemical sensor for estriol hormone detection in biological and environmental simples. J Solid State Electrochem 22:1431–1438
Vieira AP, Santana SAA, Bezerra CWB, Silva HAS, Chaves JAP, Melo JCP, da Silva Filho EC, Airoldi C (2011) Removal of textile dyes from aqueous solution by babassu coconut epicarp (Orbignya speciosa). Chem Eng J 173:334–340
Noked M, Soffer A, Aurbach D (2011) The electrochemistry of activated carbonaceous materials: past, present, and future. J Solid State Electrochem 15:1563–1578
Gangulibabu BD, Kalaiselvi N (2013) Comparison of corn starch-assisted sol–gel and combustion methods to prepare LiMn x Co y Ni z O 2 compounds. J Solid State Electrochem 17:9–17
Liew C-W, Ramesh S, Ramesh K, Arof AK (2012) Preparation and characterization of lithium ion conducting ionic liquid-based biodegradable corn starch polymer electrolytes. J Solid State Electrochem 16:1869–1875
Teoh KH, Ramesh S, Arof AK (2012) Investigation on the effect of nanosilica towards corn starch–lithium perchlorate-based polymer electrolytes. J Solid State Electrochem 16:3165–3170
Zhang Y, Jia M, Yu J, Fan J, Wang L, Zou Y, Zhao Y (2016) A tunable hierarchical porous carbon from starch pretreated by calcium acetate for high performance supercapacitors. J Solid State Electrochem 20:733–741
Zhao Q, Ma Q, Pan F, Wang Z, Yang B, Zhang J, Zhang J (2016) Facile synthesis of nitrogen-doped carbon nanosheets as metal-free catalyst with excellent oxygen reduction performance in alkaline and acidic media. J Solid State Electrochem 20:1469–1479
Fromm J (2006) Long-distance electrical signaling and physiological functions in higher plants. In Volkov AG (ed) (2006) Plant electrophysiology theory and methods. Springer, Berlin-Heidelberg
Simons P (1992) The action plant. Movement and nervous behaviour in plants. Blackwell, Oxford
Gustin MC, Zhou XL, Martinac B, Kung C (1988) A mechanosensitive ion channel in the yeast plasma membrane. Science 242:762–765
Hormann G (1898) Studien uber die Protoplasmastromung bei den Characaean. Fischer, Jena
Fromm J, Lautner S (2005) Characteristics and functions of phloem-transmitted electrical signals in higher plants. In: Baluska F, Mancuso S, Volkmann D (eds) Communication in plants—neuronal aspects of plant life. Springer, Berlin Heidelberg New York
Donohue K, Dorn L, Griffith C, Kim E, Aguilera A, Polisetty CR, Schmitt J (2005) Environmental and genetic influences on the germination of Arabidopsis thaliana in the field. Evolution 59:740–757
PubMed Google Scholar
Estrelles E, Biondi E, Galiè M, Mainardi F, Hurtado A, Soriano P (2015) Aridity level, rainfall pattern and soil features as key factors in germination strategies in salt-affected plant communities. J Arid Environ 117:1–9
Estrelles E, Prieto-Mossi J, Escribá MC, Ferrando I, Ferrer-Gallego P, Laguna E, Ibars AM, Soriano P (2018) Hydroperiod length as key parameter controlling seed strategies in Mediterranean salt marshes: The case of Halopeplis amplexicaulis . Flora 249:124–132
O’Byrne KJ, Dalgleish AG (2001) Chronic immune activation and inflammation as the cause of malignancy. Br J Cancer 85:473–483
Article PubMed PubMed Central Google Scholar
Valko M, Leibfritz D, Moncol J, Cronin MTD, Mazurand M, Telser J (2007) Free radicals and antioxidants in normal physiological functions and human disease. Int J Biochem Cell Biol 39:44–84
Hilleary R, Gilroy S (2018) Systemic signaling in response to wounding and pathogens. Curr Plant Biol 43:57–62
Doménech-Carbó A, Montoya N, Soriano P, Estrelles A (2018) An electrochemical analysis suggests role of gynodioecy in adaptation to stress in Cortaderia selloana . Curr Plant Biol 16:9–14
Doménech-Carbó A, Ibars AM, Prieto-Mossi J, Estrelles E, Scholz F, Cebrián-Torrejón G, Martini M (2015) Electrochemistry-based chemotaxonomy in plants using the voltammetry of microparticles methodology. New J Chem 39:7421–7428
Doménech-Carbó A, Ibars AM, Prieto-Mossi J, Estrelles E, Doménech-Carbó MT, Ortiz-Miranda AS, Lee Y (2017) Access to phylogeny from voltammetric fingerprints of seeds: the Asparagus case. Electroanalysis 29:643–650
Zhang SD, Soltis DE, Yang Y, Li DZ, Yi TS (2011) Multi-gene analysis provides a well-supported phylogeny of Rosales. Mol Phylogenet Evol 60:21–28
Fu L, Zheng Y, Zhang P, Zhu J, Zhang H, Zhang L, Su W (2018) Embedding leaf tissue in graphene ink to improve signals in electrochemistry-based chemotaxonomy. Electrochem Commun 92:39–42
Fu L, Zheng Y, Zhang P, Zhang H, Zhuang W, Zhang H, Wang A, Su W, Yu J, Lin C-T (2018) Enhanced electrochemical voltammetric fingerprints for plant taxonomic sensing. Biosens Bioelectron 120:102–107
Ortiz-Miranda AS, König P, Kahlert H, Scholz F, Osete-Cortina L, Doménech-Carbó MT, Doménech-Carbó A (2016) Voltammetric analysis of Pinus needles with physiological, phylogenetic and forensic applications. Anal Bioanal Chem 408:4943–4952
Muñiz-Calvo S, Guillamón JM, Dominguez I, Doménech-Carbó A (2017) Detecting and monitoring the production of melatonin and other related indole compounds in different Saccharomyces strains by solid state electrochemical techniques. Food Anal Methods 10:1408–1418
Doménech-Carbó A, Cebrián_Torrejón G, Lops-Souto A, de Moraes, MM, Jorge-Kato K, Fechine-TavaresJ, Barbosa-Filho JM (2015) Electrochemical ecology: VIMP monitoring of plant defense against external stressors. RSC Advances 5:61006–61011
Hager A, Helmle M (1981) Properties of an ATP-fueled, Cl-dependent proton pump localizad in membranes of microsomal vesicles from maize coleoptiles. Z Naturforsch 36c:997–1008
Admon AB, Jacoby B, Goldsmith EE (1981) Some characteristics of the Mg-ATPase of isolated red beet vacuoles. Plant Sci Lett 22:89–96
Walker RR, Leigh RA (1981) Characterisation of a salt-stimulated ATPase activity associated with vacuoles isolated from storage roots of red beet ( Beta vulgaris L.). Planta 153:140–149
DuPont FM, Bennett AB, Spanswick RM (1982) Localization of a proton-translocating ATPase on sucrose gradients. Plant Physiol 70:1115–1119
Mettler IJ, Mandala S, Taiz L (1982) Characterization of in vitro proton pumping by microsomal vesicles isolated from corn coleoptiles. Plant Physiol 70:1738–1742
Sze H (1985) H+-translocating ATPases: advances using membrane vesicles. Annu Rev Plant Physiol 36:175–208
Sze H, Liang F, Hwang I, Curran AC, Harper JF (2000) Diversity and regulation of plant Ca 2+ pumps: insights from expression in yeast. Annu Rev Plant Physiol Plant Mol Biol 51:433–462
Jasinski M, Ducos E, Martinoia E, Boutry M (2003) The ATP-binding cassette transporters: structure, function, and gene family comparison between rice and Arabidopsis . Plant Physiol 131:1169–1177
Churchill KA, Holoway B, Sze H (1983) Separation of two types of electrogenic H + -pumping ATPases from oat roots. Plant Physiol 73:921–928
De Michelis MI, Pugliarello MC, Rasi-Caldogno F (1983) Two distinct proton translocating ATPases are present in membrane vesicles from radish seedlings. FEBS Lett 162:85–90
Spanswick RM (2006) Electrogenic pumas, in Volkov AG, Ed (2006) Plant Electrophysiology Theory and Methods. Springer, Berlin-Heidelberg
Karny A, Zinger A, Kajal A, Shainsky-Roitman J, Schroeder A (2018) Therapeutic nanoparticles penetrate leaves and deliver nutrients to agricultural crops. Sce Rep 8: artic. 7859
Chen M, Zhou Y, Huang J, Zhu P, Peng X, Wang Y (2012) Liposome-based Delivery Systems in Plant Polysccharides. J Nanomater 2012(682545)
Agmo Hernández V, Scholz F (2006) Kinetics of the adhesion of DMPC liposomes on a mercury electrode. Effect of lamellarity, phase composition, size and curvature of liposomes and effect of the pore forming peptide mastoparan X. Langmuir 22:10723–10731
Agmo Hernández V, Hermes M, Milchev A, Scholz F (2009) The overall adhesion-spreading process of liposomes on a mercury electrode is controlled by a mixed diffusion and reaction kinetics mechanism. J Sold State Electrochem 13:639–649
Mateo EM, Gómez JV, Montoya N, Mateo-Castro R, Gimeno-Adelantado JV, Jiménez M, Doménech-Carbó A (2018) Electrochemical identification of toxigenic fungal species using solid-state voltammetry strategies. Food Chem 267:91–100
Cebrián-Torrejón G, rticu L, Ferreira ME, Rodríguez de la Cruz D, Doménech-Carbó A, Vera de Bilbao N, Rojas de Arias A, Figadère B, Poupon E, Fournet A, (2015) Harvesting canthinones: identification of the optimal seasonal point of harvest of Zanthoxylum chiloperone leaves as a source of 5-methoxycanthin-6-one. Nat Prod Res 29:2054–2058
Romero I, Domínguez I, Doménech-Carbó A, Gavara R, Escribano MI, Merodio C, Sánchez-Ballesta MT (2019) Effect of high levels of CO2 on the electrochemical articulate and the enzymatic and non-enzymatic antioxidant systems in black and white table grapes stored at 0 °C. J Science Food Agric 99:6859–6867
Cesarino I, Hümmelgen IA (2015) An additional tool towards overcoming absence of specificity of carbon nanostructure-based electrochemical sensors-application to estriol and estradiol detection and distinction. J Solid State Electrochem 19:3035–3050
Perez C, Fábio RS, Codognoto L (2015) Voltammetric determination of 17α ethinylestradiol hormone in supply dam using BDD electrode. J Solid State Electrochem 20:2471–2478
Brocenschi RF, Rocha-Filho RC, Li L, Swain GM (2014) Comparative electrochemical response of estrone at glassy-carbon, nitrogen-containing tetrahedral amorphous carbon and boron-doped diamond thin-film electrodes. J Electroanal Chem 712:207–214
Doménech-Carbó A, Doménech-Carbó MT, Ferragud-Adam X, Ortiz-Miranda AS, Montoya N, Pasíes-Oviedo T, Peiró-Ronda MA, Vives-Ferrándiz J, Carrión Y (2017) Identification of vegetal species in wooden objects using in situ microextraction-assisted voltammetry of microparticles. Anal Methods 9:2041–2048
Cebrián-Torrejón G, Pérez A, Montoya N, Piquero-Cilla J, Saldarriaga MS, Gutiérrez D, Sanders CJ, Machado W, Doménech-Carbó A (2019) Electrochemical characterization of mangrove sediments: a proposal of new proxies for organic matter oxidation. Appl Geochem 101:42–49
Doménech-Carbó A (2020) Voltammetry of immobilized particles for the future. J Solid State Electrochem 24:2063–2065
Download references
The work was carried out within the framework of project PID2020-113022GB-I00 which was financially supported by the Ministerio de Ciencia e Innovación and Agencia Estatal de Investigación (AEI) of the Spanish government.
Author information
Authors and affiliations.
Departament de Química Analítica, Universitat de València, Dr. Moliner, 50, 46100, Burjassot, Valencia, Spain
Antonio Doménech-Carbó
You can also search for this author in PubMed Google Scholar
Corresponding author
Correspondence to Antonio Doménech-Carbó .
Additional information
Publisher's note.
Springer Nature remains neutral with regard to jurisdictional claims in published maps and institutional affiliations.
Rights and permissions
Reprints and permissions
About this article
Doménech-Carbó, A. Electrochemistry of plants: basic theoretical research and applications in plant science. J Solid State Electrochem 25 , 2747–2757 (2021). https://doi.org/10.1007/s10008-021-05046-1
Download citation
Received : 14 July 2021
Revised : 29 August 2021
Accepted : 31 August 2021
Published : 13 September 2021
Issue Date : December 2021
DOI : https://doi.org/10.1007/s10008-021-05046-1
Share this article
Anyone you share the following link with will be able to read this content:
Sorry, a shareable link is not currently available for this article.
Provided by the Springer Nature SharedIt content-sharing initiative
- Electrochemistry
- Biochemistry
- Chemoecology
- Find a journal
- Publish with us
- Track your research

- News Releases
IIT researchers show how plants can generate electricity to power LED light bulbs
In Advanced Functional Materials, the study shows that single plant leaves can generate more than 150 volts; a 'hybrid tree' made of natural and artificial leaves can act as an innovative 'green' electric generator
Istituto Italiano di Tecnologia - IIT
image: The hybrid plant is made of natural and artificial leaves. When wind blows into the plant and moves the leaves, the 'hybrid tree' produces electricity. view more
Credit: IIT-Istituto Italiano di Tecnologia
Sustainable energy sources, which are pollution free and environmentally friendly, are one of the key challenges of world's future society. The interdisciplinary team of roboticists and biologists at IIT-Istituto Italiano di Tecnologia in Pontedera (Pisa, Italy), found that living plants can help with electricity. Fabian Meder, Barbara Mazzolai and their coworkers at IIT discovered that living plants are literally "green" power source, which may become one of future's electricity supplies that perfectly integrates in natural environments and is accessible all over the world. Researchers discovered that plants can generate, by a single leaf, more than 150 Volts, enough to simultaneously power 100 LED light bulbs. Researchers also showed that an "hybrid tree" made of natural and artificial leaves can act as an innovative "green" electrical generator converting wind into electricity.
Results are published on Advanced Functional Materials .
The research team is based at Center for Micro-Bio Robotics (CMBR) of IIT in Pontedera (Pisa, Italy), coordinated by Barbara Mazzolai, and their goal is to perform advanced research and to develop innovative methodologies, robotic technologies and new materials, inspired by the natural world. Bio-inspired approaches can therefore help to develop robots and technologies that are more suitable for unstructured environments than today's solutions. In 2012 Barbara Mazzolai coordinated the EU funded project Plantoid , which brought to the realization of the first plant robot in the world. In this last study, the research team studied plants and showed that leaves can create electricity when they are touched by a distinct material or by the wind.
Certain leaf structures are capable to convert mechanical forces applied at the leaf surface into electrical energy, because of the specific composition that most plant leaves naturally provide. In detail, the leaf is able to gather electric charges on its surface due to a process called contact electrification. These charges are then immediately transmitted into the inner plant tissue. The plant tissue acts similar to a "cable" and transports the generated electricity to other parts of the plant. Hence, by simply connecting a "plug" to the plant stem, the electricity generated can be harvested and used to power electronic devices. IIT's researchers show that the voltage generated by a single leaf may reach to more than 150 Volts, enough to simultaneously power 100 LED light bulbs each time the leaf is touched.
In the article, researchers additionally describe for the first time how this effect can be used to convert wind into electricity by plants. Therefore, researchers modified a Nerum oleander tree with artificial leaves that touch the natural N. oleander leaves. When wind blows into the plant and moves the leaves, the "hybrid tree" produces electricity. The electricity generated increases the more leaves are touched. Consequently, it can be easily up-scaled by exploiting the whole surface of the foliage of a tree or even a forest.
The study is a first essential step for a new project that Barbara Mazzolai will coordinate in 2019, the European-funded project Growbot whose aim is to realize bioinspired robots that implement plant-like growing motions. The new robots will be then partly powered by the new plant-derived energy source, showing that plants may become one of future's electricity supplies, accessible all over the world.
Advanced Functional Materials
10.1002/adfm.201806689
Disclaimer: AAAS and EurekAlert! are not responsible for the accuracy of news releases posted to EurekAlert! by contributing institutions or for the use of any information through the EurekAlert system.
Additional Multimedia
A novel electricity generation with green technology by Plant-e from living plants and bacteria: A natural solar power from living power plant
Ieee account.
- Change Username/Password
- Update Address
Purchase Details
- Payment Options
- Order History
- View Purchased Documents
Profile Information
- Communications Preferences
- Profession and Education
- Technical Interests
- US & Canada: +1 800 678 4333
- Worldwide: +1 732 981 0060
- Contact & Support
- About IEEE Xplore
- Accessibility
- Terms of Use
- Nondiscrimination Policy
- Privacy & Opting Out of Cookies
A not-for-profit organization, IEEE is the world's largest technical professional organization dedicated to advancing technology for the benefit of humanity. © Copyright 2024 IEEE - All rights reserved. Use of this web site signifies your agreement to the terms and conditions.

Home → Research
Electricity from trees
You're not going to power too many devices with electricity from trees. But it is a very interesting phenomenon.
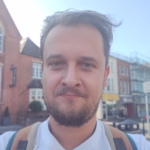
Researchers have figured out a way to ‘plug’ into electrical power generated by trees. Although this isn’t a practical way to power up electric circuits, it still offers intriguing insights into the biochemical processes in trees — and maybe about electricity itself.
Tree electricity is not like potato electricity
It has been a well known fact for years that plants can conduct electricity (humans can too, take care kids). Now scientists from MIT found out just how much they can pack up: 200 millivolts of electrical power (=0.2 volts). To put it into perspective, that’s 25 times less than the 5 volts that power radios and other small devices.
The lemon and potato battery experiments are already notorious, but this is something else. The mechanism through which researchers can harvest electricity from trees is completely different.
“We specifically didn’t want to confuse this effect with the potato effect, so we used the same metal for both electrodes,” said Babak Parviz, a professor of electrical engineering at Washington University and co-author of the study.
After spending months surveying trees and analyzing their current flow, Parviz and colleagues found that big leaf maple trees can generate generated a steady voltage.
However, in order to become practical, a much higher voltage is necessary. So researchers built a boost converter capable of picking up really little voltages and storing them and then producing a greater output.
With this device, researchers were able to generate an output voltage of 1.1 volts. Already, this is enough to power some sensors. For instance, some environmental sensors that track temperature or humidity.
Essentially, the tree would be powering the sensors with its own electricity.
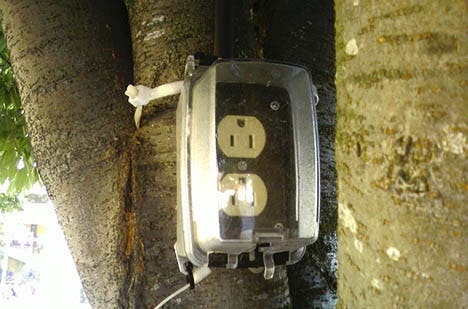
The full study will be published in the upcoming issue of the Institute of Electrical and Electronics Engineers’ Transactions on Nanotechnology . It’s not really as practical as other options, but it could prove to be quite significant, especially for different types of sensors.
“Normal electronics are not going to run on the types of voltages and currents that we get out of a tree.” Parviz said. “As new generations of technology come online, I think it’s warranted to look back at what’s doable or what’s not doable in terms of a power source.”
But this isn’t the only time researchers drew electricity from trees.
Fabian Meder, Barbara Mazzolai, and their coworkers at Istituto Italiano di Tecnologia in Pontedera (Pisa, Italy), also harvested electricity. This team showed that in some instances, plants can generate, by a single leaf, more than 150 Volts when you touch them.
Researchers also describe how this effect can be used to transform wind into electricity through plants. Yet again, this isn’t going to be significant in the grand scheme of things, for now, but it shows how plants and electricity interact together in unforeseen ways.
Who knows, maybe in the future, we can even find a way to use this. But for now, this is not meant to be used by humans. In fact, researchers suspect it can be used as a tool to look at a tree’s health and its biology.
“It’s not exactly established where these voltages come from. But there seems to be some signaling in trees, similar to what happens in the human body but with slower speed,” Parviz said. “I’m interested in applying our results as a way of investigating what the tree is doing. When you go to the doctor, the first thing that they measure is your pulse. We don’t really have something similar for trees.”
Was this helpful?
Related posts.
- New imaging method reveals stunning methods of brain connections
- Highest Silicon Solar Cell Efficiency Ever Reached
- Large Hadron Collider hints at infant Universe
- LHC produces first results
- Editorial Policy
- Privacy Policy and Terms of Use
- How we review products
© 2007-2023 ZME Science - Not exactly rocket science. All Rights Reserved.
- Science News
- Environment
- Natural Sciences
- Matter and Energy
- Quantum Mechanics
- Thermodynamics
- Periodic Table
- Applied Chemistry
- Physical Chemistry
- Biochemistry
- Microbiology
- Plants and Fungi
- Planet Earth
- Earth Dynamics
- Rocks and Minerals
- Invertebrates
- Conservation
- Animal facts
- Climate change
- Weather and atmosphere
- Diseases and Conditions
- Mind and Brain
- Food and Nutrition
- Anthropology
- Archaeology
- The Solar System
- Asteroids, meteors & comets
- Astrophysics
- Exoplanets & Alien Life
- Spaceflight and Exploration
- Computer Science & IT
- Engineering
- Sustainability
- Renewable Energy
- Green Living
- Editorial policy
- Privacy Policy
Plants at Home
Can Plants Conduct Electricity?
For every electrical circuit to work, the current must be able to flow through the various components. So, what happens if we use a plant as an electrical component?
Can plants conduct electricity?
No, plants in their natural state cannot conduct electricity. However, if the conditions are altered or conductive elements are used in the plant, they can conduct electricity. While this shows that plants can be used to conduct electricity, we must remember that it can only be done artificially.
Let us explore how plants can be made to conduct electricity.
Electrical Conductor
An electrical conductor is a material through which electrical current can flow easily. It is the opposite of an electrical insulator, which is a material that prevents the flow of electricity through it. An example of a good conductor is copper or gold, which offers minimal resistance. Hence, copper or gold is generally used in circuits.
However, the question of whether plants can be used as a conductor is still a question that has not been completely answered by science.
Scientific Research
In 2015, Swedish researchers successfully showed that electricity could be circulated through a plant. It was done by injecting a conductive gel into the plant. It enabled the plant to successfully conduct electricity and also opened certain possibilities. It could be used to manage plant growth as well as produce electricity through photosynthesis.
Conductivity of Plants
Plants are essentially made up of around 80 to 95% water. We know that water can be used as a conductor. It contains sediments and minerals that ionize the water molecules and allow electricity to be conducted very well through it. Thus, it makes plants good conductors of electricity.
Green Energy
With global warming taking its toll the production of green and clean energy has become one of the primary concerns. While electricity in itself is clean, the means to produce it, such as coal, oil, or nuclear power tend to cause serious environmental hazards.
While science has rapidly progressed to develop cleaner sources of electricity production such as solar panels, hydroelectricity, and wind turbines, they have only been used on a small scale. So, the development of energy through plants could be a game-changer due to the abundant presence of plants on earth.
Bionic Plants
The Swedish researchers successfully developed a bionic rose that could conduct electricity. It was done by creating a water-soluble polymer called PEDOT-S, which was integrated into the plant by using the polymer dissolved water for watering the plant. It helped the researchers create a circuit, that used the xylem, veins, and leaves of the plant to conduct electricity. It was the first conductive plant in the world.
Final Words
Thus, we can see that no plant has the natural ability to conduct. However, the development of the bionic rose has opened up the possibility of being able to capture the energy of the plant, that is generated through photosynthesis and to use it to produce the required green and clean energy. However, the commercial viability of this project is yet to be explored.
Related posts:
- Do Plants Have Feelings?
- Do Plants Have DNA?
- Do Plants Have Organs?
- Do Plant Cells Have Centrioles?
- Do Plant Cells Have Mitochondria?
- Do Plant Cells Have Chloroplasts?
- What Do Plant Cells Have That Animal Cells Do Not?
- Do Plant Cells Have Vacuoles?
Leave a Comment Cancel reply
Save my name, email, and website in this browser for the next time I comment.
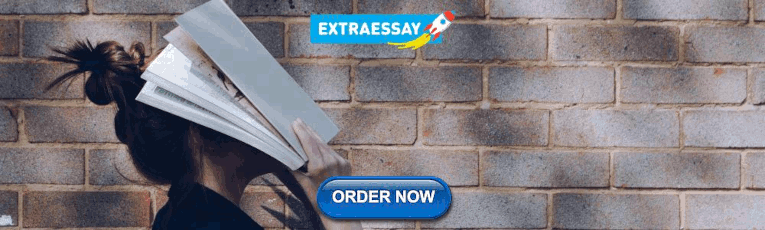
COMMENTS
Researchers discovered that plants can generate, by a single leaf, more than 150 Volts, enough to simultaneously power 100 LED light bulbs. Researchers also showed that an "hybrid tree" made of ...
Based on th e problems and considered the i deas, it is necessary to study the potential of electrical. energy from living plants in Indonesian forests, specifically in urban forest parks. This ...
Now it appears that living plants can also contribute to energy production. In a plant microbial fuel cell, living plants work together with micro-organisms to create electricity. This results in clean and renewable energy, while the plant remains alive. The first practical tests have been very promising. In projects supported by the EU and ...
One company has found a way to harvest electrons deposited by plants into the soil beneath them. But new research from Finland looks at tapping plants' energy directly by turning their internal ...
Research on the generation of electrical energy from living plants has been widely carried out and gives satisfying results. In this study, the living plants studied were urban tropical forest plants so that the electricity generated could be used for lighting or sources of charging batteries for electronic devices or electric vehicles.
But by collecting electrons naturally transported within plant cells, scientists can generate electricity as part of a "green," biological solar cell. Now, researchers reporting in ACS Applied Materials & Interfaces have, for the first time, used a succulent plant to create a living "bio-solar cell" that runs on photosynthesis.
3.1 Electric fields affect plant growth. For centuries, scientists have explored how electric fields affect plants. A summary of these studies is given in Table 1.In Karl Lemström (), they investigated the effects of electric fields using large-scale experiments for the first time.He questioned whether plants growing in the Arctic, where the Earth's electric field is stronger than in major ...
This research was conducted under the auspices of the Graduate School SENSE (Socio-Economic and Natural Sciences of the Environment). Electricity generation by living plants in a plant microbial fuel cell Ruud Timmers Thesis submitted in fulfillment of the requirements for the degree of doctor
Nature Reviews Materials 7 , 255 ( 2022) Cite this article. Photosynthetic cells, if connected to electrodes, can be used to generate electricity. However, designing efficient cell-electrode ...
The chemical reaction by which plants produce complex food molecules (sugars) requires an energy input (i.e., from sunlight) to occur. In this reaction, carbon dioxide and water combine to form carbon-based organic molecules and release oxygen. (secondary to MS-LS1-6) Science and Engineering Practices. Modeling in 6-8 builds on K-5 ...
The rest is excreted via the roots. Around the roots, bacteria feed on the organic matter and they release electrons. If you're able to harvest the electrons into an electrode, you can couple the first electrode to a counter-electrode and build an electrical circuit, like in a battery. The electrons flow back into the natural system via the ...
Electrochemistry of plants can be viewed as a multifaceted research fields including electrophysiological studies around signaling in plants and those associated with the interaction of plants with the environment. Apart from botanic, this research can be implemented into agriculture, food chemistry, ecology, climatology, and other fields with a variety of applications. Vegetal matter can also ...
Although experimental details often were incomplete, positive effects of electricity on plant growth have been reported over the past 200 years (Tattar and Blanchard, 1976).An overview of the effects of strong electric and magnetic fields on plants is given in Table 1.As such, Lemström (1904) was the first, who demonstrated the stimulating effects of natural electrostatic fields on plants ...
This research was conducted to explore alternative energy sources that are green, namely the source of electrical energy from living plants. Research on the generation of electrical energy from living plants has been widely carried out and gives satisfying results. In this study, the living plants studied were
IIT researchers show how plants can generate electricity to power LED light bulbs. In Advanced Functional Materials, the study shows that single plant leaves can generate more than 150 volts; a ...
The effectiveness of using electric fields to stimulate crop growth, known as electroculture, is far from established, despite being tested in Europe, the US and China. A "golden age" is dawning for the technology, one Chinese scientist told New Scientist in 2019. Now, Jianjun Luo at the Chinese Academy of Sciences in Beijing and his ...
electricity in l iving-plant fuel cell, or LFC [13-14]. This. paper is intended to model the electricity generation. in LFC based on the electrochemistry mechanisms. In. the experiment presen ted ...
One relatively recent discovery related to the triboelectric effect is that it applies to living materials like plants. Specifically, the tissue within plant leaves can conduct electricity, while ...
Every country requires sufficient amount of electricity for their development. In fact, the world needs a renewable, an efficient and sustainable energy production to safeguard our future earth. We documented it, for the people around the world to know about this fascinating technology of young innovative company, Plant-e bringing creative projects to life. It is based on, living plants in ...
Tree electricity is not like potato electricity. It has been a well known fact for years that plants can conduct electricity (humans can too, take care kids). Now scientists from MIT found out ...
Almost all of a plant's life activities involve electrochemical reactions. Plant electrical parameters respond quickly to environmental changes and are closely related to physiological activities. In this study, the theoretical intrinsic relationships between clamping force and leaf impedance (Z) or capacitive reactance (Xc) and capacitance (C) were revealed as 3-parameter exponential decay ...
No, plants in their natural state cannot conduct electricity. However, if the conditions are altered or conductive elements are used in the plant, they can conduct electricity. While this shows that plants can be used to conduct electricity, we must remember that it can only be done artificially. Let us explore how plants can be made to conduct ...
This study aimed to a field experiment that was carried out at the Field Experimental Station of the Institute of Plant Protection—National Research Institute in Winna Góra, Poland, during the 2022/2023 season to evaluate the potential of Lamium album (L. album) flower extract as a foliar spray against mycotoxigenic fungi in two winter wheat ...
High-Temperature Materials Science Experiment Cabinet on the Chinese Space Station is mainly used to carry out experimental research related to high-temperature materials science in microgravity. It is equipped with an X-ray transmission imaging module, which is applied to realize transmission imaging of material samples under microgravity. However, the X-ray light source is far away from the ...