Can coastal cities turn the tide on rising flood risk?
Climate change is increasing the destructive power of flooding from extreme rain and rising seas and rivers. Many cities around the world are exposed. Strong winds during storms and hurricanes can drive coastal flooding through storm surge. As hurricanes and storms become more severe, surge height increases. Changing hurricane paths may shift risk to new areas. Sea-level rise amplifies storm surge and brings in additional chronic threats of tidal flooding. Pluvial and riverine flooding becomes more severe with increases in heavy precipitation. Floods of different types can combine to create more severe events known as compound flooding. With warming of 1.5 degrees Celsius , 11 percent of the global land area is projected to experience a significant increase in flooding, while warming of 2.0 degrees almost doubles the area at risk.
When cities flood, in addition to often devastating human costs, real estate is destroyed, infrastructure systems fail, and entire populations can be left without critical services such as power, transportation, and communications. In this case study we simulate floods at the most granular level (up to two-by-two-meter resolution) and explore how flood risk may evolve for Ho Chi Minh City (HCMC) and Bristol (See sidebar, “An overview of the case study analysis”). Our aim is to illustrate the changing extent of flooding, the landscape of human exposure, and the magnitude of societal and economic impacts.
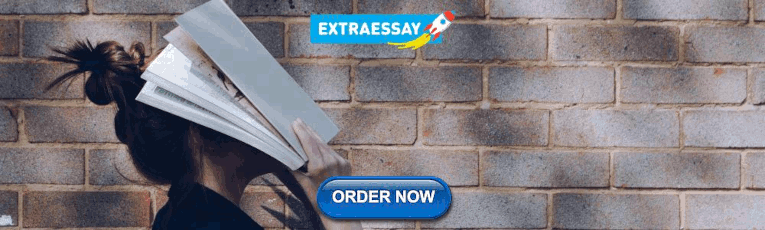
An overview of the case study analysis
In Climate risk and response: Physical hazards and socioeconomic impacts , we measured the impact of climate change by the extent to which it could affect human beings, human-made physical assets, and the natural world. We explored risks today and over the next three decades and examined specific cases to understand the mechanisms through which climate change leads to increased socioeconomic risk.
In order to link physical climate risk to socioeconomic impact, we investigated cases that illustrated exposure to climate change extremes and proximity to physical thresholds. These cover a range of sectors and geographies and provide the basis of a “micro-to-macro” approach that is a characteristic of McKinsey Global Institute research. To inform our selection of cases, we considered over 30 potential combinations of climate hazards, sectors, and geographies based on a review of the literature and expert interviews on the potential direct impacts of physical climate hazards. We found these hazards affect five different key socioeconomic systems: livability and workability, food systems, physical assets, infrastructure services, and natural capital.
We ultimately chose nine cases to reflect these systems and to represent leading-edge examples of climate change risk. Each case is specific to a geography and an exposed system, and thus is not representative of an “average” environment or level of risk across the world. Our cases show that the direct risk from climate hazards is determined by the severity of the hazard and its likelihood, the exposure of various “stocks” of capital (people, physical capital, and natural capital) to these hazards, and the resilience of these stocks to the hazards (for example, the ability of physical assets to withstand flooding). We typically define the climate state today as the average conditions between 1998 and 2017, in 2030 as the average between 2021 and 2040, and in 2050 between 2041 and 2060. Through our case studies, we also assess the knock-on effects that could occur, for example to downstream sectors or consumers. We primarily rely on past examples and empirical estimates for this assessment of knock-on effects, which is likely not exhaustive given the complexities associated with socioeconomic systems. Through this “micro” approach, we offer decision makers a methodology by which to assess direct physical climate risk, its characteristics, and its potential knock-on impacts.
Climate science makes extensive use of scenarios ranging from lower (Representative Concentration Pathway 2.6) to higher (RCP 8.5) CO 2 concentrations. We have chosen to focus on RCP 8.5, because the higher-emission scenario it portrays enables us to assess physical risk in the absence of further decarbonization. (We also choose a sea-level rise scenario for one of our cases that is consistent with the RCP 8.5 trajectory). Such an "inherent risk" assessment allows us to understand the magnitude of the challenge and highlight the case for action. For a detailed description of the reason for this choice see the technical appendix of the full report.
Our case studies cover each of the five systems we assess to be directly affected by physical climate risk, across geographies and sectors. While climate change will have an economic impact across many sectors, our cases highlight the impact on construction, agriculture, finance, fishing, tourism, manufacturing, real estate, and a range of infrastructure-based sectors. The cases include the following:
- For livability and workability, we look at the risk of exposure to extreme heat and humidity in India and what that could mean for that country’s urban population and outdoor-based sectors, as well as at the changing Mediterranean climate and how that could affect sectors such as wine and tourism.
- For food systems, we focus on the likelihood of a multiple-breadbasket failure affecting wheat, corn, rice, and soy, as well as, specifically in Africa, the impact on wheat and coffee production in Ethiopia and cotton and corn production in Mozambique.
- For physical assets, we look at the potential impact of storm surge and tidal flooding on Florida real estate and the extent to which global supply chains, including for semiconductors and rare earths, could be vulnerable to the changing climate.
- For infrastructure services, we examine 17 types of infrastructure assets, including the potential impact on coastal cities such as Bristol in England and Ho Chi Minh City in Vietnam.
- Finally, for natural capital, we examine the potential impacts of glacial melt and runoff in the Hindu Kush region of the Himalayas; what ocean warming and acidification could mean for global fishing and the people whose livelihoods depend on it; as well as potential disturbance to forests, which cover nearly one-third of the world’s land and are key to the way of life for 2.4 billion people.
We chose these cities for the contrasting perspectives they offer: Ho Chi Minh City in an emerging economy, Bristol in a mature economy; Ho Chi Minh City in a regular flood area, Bristol in an area developing a significant flood risk for the first time in a generation.
We find the metropolis of Ho Chi Minh City can survive its flood risk today, but its plans for rapid infrastructure expansion and continued economic growth could be incompatible with an increase in risk. The city has a wide range of adaptation options at its disposal but no silver bullet.
In the much smaller city of Bristol, we find a risk of flood damages growing from the millions to the billions, driven by high levels of exposure. The city has fewer adaptation options at its disposal, and its biggest challenge may be building political and financial support for change.
How significant are the flood risks facing Ho Chi Minh City and what can the city do?
Flooding is a common part of life in Ho Chi Minh City. This includes flooding from monsoonal rains, which account for about 90 percent of annual rainfall, tidal floods and storm surge from typhoons and other weather events. Of the city’s 322 communes and wards, about half have a history of regular flooding with 40 to 45 percent of land in the city less than one meter above sea level.
In our analysis, we quantify the possible impact on the city as floods hit real estate and infrastructure assets. 1 Flood modeling and expert guidance were provided by an academic consortium of Institute for Environmental Studies, Vrije Universiteit Amsterdam, and Center of Water Management and Climate Change, Vietnam National University. Infrastructure assets covered include both those currently available and those under construction, planned, or speculated. Knock-on effects are adjusted for estimates of economic and population growth. We simulate possible 1 percent probability flooding scenarios for the city for three periods: today, 2050, and a longer-term scenario of 180 centimeters of sea-level rise, which some infrastructure assets built by 2050 may experience as a worse-case in their lifetime (Exhibit 1).
- Today: We estimate that 23 percent of the city could flood, and a range of existing assets would be taken offline; infrastructure damage may total $200 million to $300 million. Knock-on effects would be significant, and we estimate could total a further $100 million to $400 million. Real estate damage may total $1.5 billion.
- 2050: A flood with the same probability in 30 years’ time would likely do three times the physical damage and deliver 20 times the knock-on effects. We estimate that 36 percent of the city becomes flooded. In addition, many of the 200 new infrastructure assets are planned to be built in flooded areas. As a result, the damage bill would grow, totaling $500 million to $1 billion. Increased economic reliance on assets would amplify knock-on effects, leading to an estimated $1.5 billion to $8.5 billion in losses. An additional $8.5 billion in real estate damages could occur.
- A 180 centimeters sea-level rise scenario: A 1 percent probability flood in this scenario may bring three times the extent of flood area. About 66 percent of the city would be underwater, driven by a large western area that suddenly pass an elevation threshold. Under this scenario, damage is critical and widespread, totaling an estimated $3.8 billion to $7.3 billion. Much of the city’s functionality may be shut down, with knock-on effects costing $6.4 billion to $45.1 billion. Real estate damage could total $18 billion.
While “tail” events may suddenly break systems and cause extraordinary impact, extreme floods will be infrequent. Intensifying chronic events are more likely to have a greater effect on the economy, with a mounting annual burden over time. We estimate that intensifying regular floods may rise from about 2 percent today to about 3 percent of Ho Chi Minh City’s GDP annually by 2050 (Exhibit 2).
Ho Chi Minh City has time to adapt, and the city has many options to avert impacts because it is relatively early in its development journey. As less than half of the city’s major infrastructure needed for 2050 exists today, many of the potential adaptation options could be highly effective. We outline three key steps:
- Better planning to reduce exposure and risk
- Investing in adaptation through hardening and resilience
- Financial mobilization to mitigate impacts on lower-income populations
For additional details on these actions, download the case study, Can coastal cities turn the tide on rising flood risk? (PDF–4MB).
Could Bristol’s flood risk grow from a problem to a crisis by 2065?
Bristol is facing a new flood risk. The river Avon, which runs through the city, has the second largest tidal range in the world, yet it has not caused a major flood since 1968, when sea levels were lower, and the city was smaller and less developed. During very high tides, the Avon becomes “tide locked” and limits/restricts land drainage in the lower reaches of river catchment area. As a result, the city is vulnerable to combined tidal and pluvial floods, which are sensitive to both sea-level rise and precipitation increase. Both are expected to climb with climate change . While Bristol is generally hilly and most of the urban area is far from the river, the most economically valuable areas of the city center and port regions are on comparatively low-lying land.
With the city’s support, we have modeled the socioeconomic impacts of 200-year (0.5 percent probability) combined tidal and fluvial flood risk, for today and for 2065. This considers the flood defenses in existence today; some of these were built after the 1968 flood, and many assumed a static climate would exist for their lifetime (Exhibit 3).
- Today: The consequences of a major flood today in Bristol would be small but are still material. We find that the flood area would be relatively minor, with small overflows on the edges of the port area and isolated floods in the center of the city. Our model estimates that damage to the city’s infrastructure could amount to $10 million to $25 million, real estate damage to $15 million to $20 million, and knock-on effects of $20 million to $150 million.
- 2065: In contrast, by 2065, an extreme flood event could be devastating. Water would exceed the city’s flood defenses at multiple locations, hitting some of its most expensive real estate, damaging arterial transportation infrastructure, and destroying sensitive critical energy assets. Our model estimates that damages to the city’s infrastructure could amount to between $180 million and $390 million. It may also cause $160 million to $240 million of property damage. Overall, considering economic growth, knock-on effects could total $500 million to $2.8 billion, and disruptions could last weeks or months (Exhibit 4).
Unlike many small and medium-size cities, Bristol has invested in understanding this risk. It has undertaken a detailed review of how the scale of flooding in the city will change in the future under different climate scenarios. This improved understanding of the risks is an example that other cities could learn from.
However, adaptation is unlikely to be straightforward. It is difficult to imagine Bristol’s infrastructure assets being in a position more exposed to the city’s flood risk. Yet the center of the city, formed in the 1400s, cannot be shifted overnight, nor would its leafy reputation be the same today if the city had not oriented the growth of the past 20 years to harness its existing Edwardian and Victorian architecture. Unlike in Ho Chi Minh City, most of the infrastructure the city plans to have in place in 2065 has already been built.
In the immediate future, Bristol’s hands are likely largely tied, and hard adaptation may be the most viable short-term solution. In the medium term, however, Bristol may be able to act to improve resilience through measures such as investing in sustainable urban drainage that may reduce the depth and duration of an extreme flood event.
Bristol is already taking a proactive approach to adaptation. A $130 million floodwall for the defense of Avonmouth was planned to begin in late 2019. The city is still scoping out a range of options to protect the city. As an outside-in estimate, based on scaling costs to build the Thames Barrier in 1982, plus additional localized measures that might be needed, protecting the city to 2065 may cost $250 million to $500 million (roughly 0.5 to 1.5 percent of Bristol’s GVA today compared to the possible flood impact we calculate of between 2 to 9 percent of the city’s GVA in 2065). However, the actual costs will largely depend on the final approach.
Bristol has gotten ahead of the game by improving its own understanding of risk. Many other small cities are at risk of entering unawares into a new climatic band for which they and their urban areas are ill prepared. While global flood risk is concentrated in major coastal metropolises, a long tail of other cities may be equally exposed, less prepared, and less likely to bounce back.
For additional details, download the case study, Can coastal cities turn the tide on rising flood risk? (PDF–4MB).
About this case study:
In January 2020, the McKinsey Global Institute published Climate risk and response: Physical hazards and socioeconomic impacts . In that report, we measured the impact of climate change by the extent to which it could affect human beings, human-made physical assets, and the natural world over the next three decades. In order to link physical climate risk to socioeconomic impact, we investigated nine specific cases that illustrated exposure to climate change extremes and proximity to physical thresholds.
Explore a career with us
Related articles.

Climate risk and response: Physical hazards and socioeconomic impacts

Earth to CEO: Your company is already at risk from climate change

Thomas L. Friedman: The three climate changes

Search form
- Find Stories
- For Journalists
Climate change has caused billions of dollars in flood damages, according to Stanford researchers
Flooding has caused hundreds of billions of dollars in damage in the U.S. over the past three decades. Researchers found that 36 percent of the costs of flooding in the U.S. from 1988 to 2017 were a result of intensifying precipitation, consistent with predictions of global warming.
In a new study, Stanford researchers report that intensifying precipitation contributed one-third of the financial costs of flooding in the United States over the past three decades, totaling almost $75 billion of the estimated $199 billion in flood damages from 1988 to 2017.
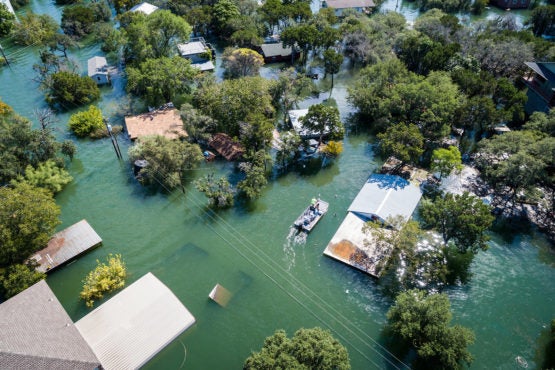
Water rescue crew searches by boat for survivors after a dangerous flooding event. In a new analysis, researchers attribute about one-third of the cost of flooding damages in the past 30 years to climate change. (Image credit: Roschetzky / iStockPhoto)
The research , published Jan. 11 in the journal Proceedings of the National Academy of Sciences , helps to resolve a long-standing debate about the role of climate change in the rising costs of flooding and provides new insight into the financial costs of global warming overall.
“The fact that extreme precipitation has been increasing and will likely increase in the future is well known, but what effect that has had on financial damages has been uncertain,” said lead author Frances Davenport, a PhD student in Earth system science at Stanford’s School of Earth, Energy & Environmental Sciences (Stanford Earth). “Our analysis allows us to isolate how much of those changes in precipitation translate to changes in the cost of flooding, both now and in the future.”
The global insurance company Munich Re calls flooding “the number-one natural peril in the U.S.” However, although flooding is one of the most common, widespread and costly natural hazards, whether climate change has contributed to the rising financial costs of flooding – and if so, how much – has been a topic of debate, including in the most recent climate change assessments from the U.S. government and the Intergovernmental Panel on Climate Change.
At the crux of that debate is the question of whether or not the increasing trend in the cost of flooding in the U.S. has been driven primarily by socioeconomic factors like population growth, housing development and increasing property values. Most previous research has focused either on very detailed case studies (for example, of individual disasters or long-term changes in individual states) or on correlations between precipitation and flood damages for the U.S. overall.
In an effort to close this gap, the researchers started with higher resolution climate and socioeconomic data. They then applied advanced methods from economics to quantify the relationship between historical precipitation variations and historical flooding costs, along with methods from statistics and climate science to evaluate the impact of changes in precipitation on total flooding costs. Together, these analyses revealed that climate change has contributed substantially to the growing cost of flooding in the U.S., and that exceeding the levels of global warming agreed upon in the United Nations Paris Agreement is very likely to lead to greater intensification of the kinds of extreme precipitation events that have been most costly and devastating in recent decades.
“Previous studies have analyzed pieces of this puzzle, but this is the first study to combine rigorous economic analysis of the historical relationships between climate and flooding costs with really careful extreme event analyses in both historical observations and global climate models, across the whole United States,” said senior author and climate scientist Noah Diffenbaugh , the Kara J Foundation Professor at Stanford Earth.
“By bringing all those pieces together, this framework provides a novel quantification not only of how much historical changes in precipitation have contributed to the costs of flooding, but also how greenhouse gases influence the kinds of precipitation events that cause the most damaging flooding events,” Diffenbaugh added.
The researchers liken isolating the role of changing precipitation to other questions of cause and effect, such as determining how much an increase in minimum wage will affect local employment, or how many wins an individual player contributes to the overall success of a basketball team. In this case, the research team started by developing an economic model based on observed precipitation and monthly reports of flood damage, controlling for other factors that might affect flooding costs like increases in home values. They then calculated the change in extreme precipitation in each state over the study period. Finally, they used the model to calculate what the economic damages would have been if those changes in extreme precipitation had not occurred.
“This counterfactual analysis is similar to computing how many games the Los Angeles Lakers would have won, with and without the addition of LeBron James, holding all other players constant,” said study co-author and economist Marshall Burke , an associate professor of Earth system science.
Applying this framework, the research team found that – when totaled across all the individual states – changes in precipitation accounted for 36 percent of the actual flooding costs that occurred in the U.S. from 1988 to 2017. The effect of changing precipitation was primarily driven by increases in extreme precipitation, which have been responsible for the largest share of flooding costs historically.
“What we find is that, even in states where the long-term mean precipitation hasn’t changed, in most cases the wettest events have intensified, increasing the financial damages relative to what would have occurred without the changes in precipitation,” said Davenport, who received a Stanford Interdisciplinary Graduate Fellowship in 2020.
The researchers emphasize that, by providing a new quantification of the scale of the financial costs of climate change, their findings have implications beyond flooding in the U.S.
“Accurately and comprehensively tallying the past and future costs of climate change is key to making good policy decisions,” said Burke. “This work shows that past climate change has already cost the U.S. economy billions of dollars, just due to flood damages alone.”
The authors envision their approach being applied to different natural hazards, to climate impacts in different sectors of the economy and to other regions of the globe to help understand the costs and benefits of climate adaptation and mitigation actions.
“That these results are as robust and definitive as they are really advances our understanding of the role of historical precipitation changes in the financial costs of flooding,” Diffenbaugh said. “But, more broadly, the framework that we developed provides an objective basis for estimating what it will cost to adapt to continued climate change and the economic value of avoiding higher levels of global warming in the future.”
Diffenbaugh is also the Kimmelman Family Senior Fellow at the Stanford Woods Institute for the Environment and an affiliate of the Precourt Institute for Energy . Burke is also deputy director of the Center on Food Security and the Environment and a fellow at the Stanford Woods Institute, the Freeman Spogli Institute for International Studies and the Stanford Institute for Economic Policy Research .
To read all stories about Stanford science, subscribe to the biweekly Stanford Science Digest .
- 0 Shopping Cart
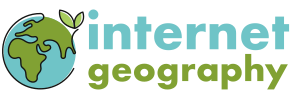
UK Floods Case Study November 2019
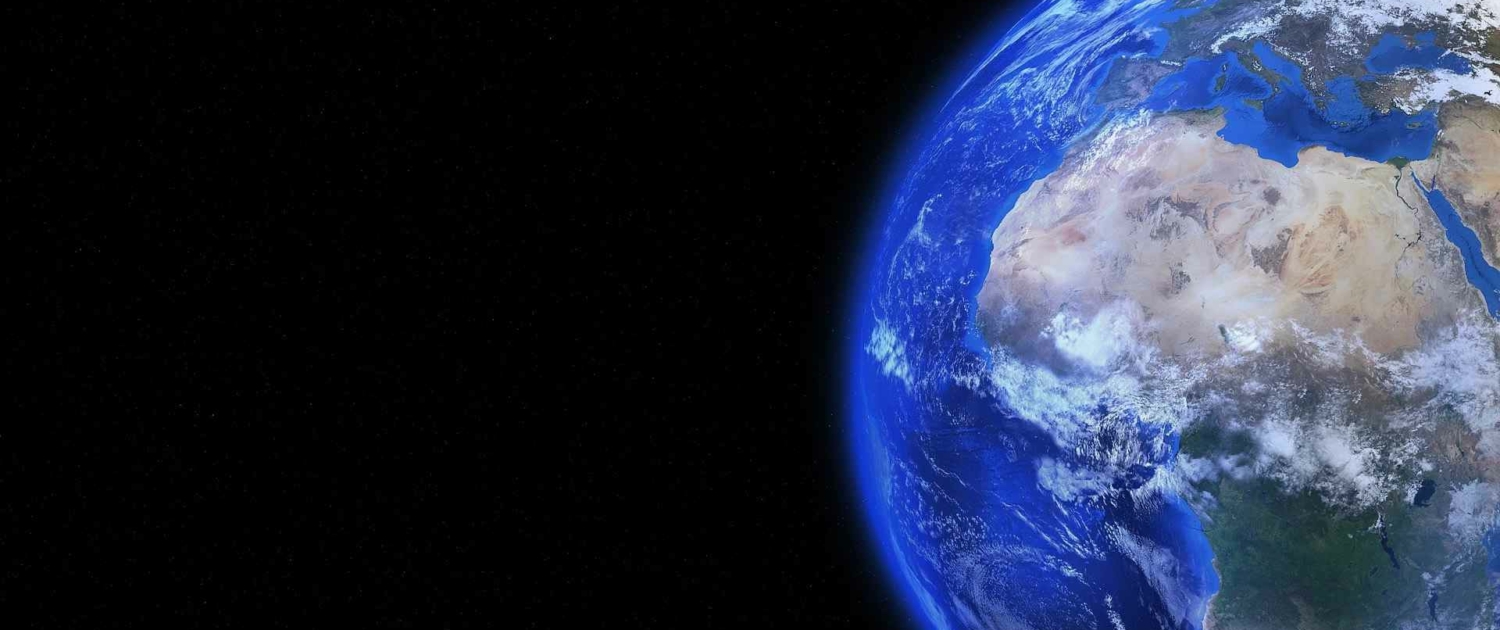
The UK experienced an extreme weather event in November 2019 when exceptionally heavy rainfall caused flooding in parts of the UK. Heavy downpours across large parts of northern England led to surface water and river flooding in parts of Yorkshire, Nottinghamshire, Greater Manchester, Derbyshire and Lincolnshire.
According to the Met Office, on Thursday 7th November 2019 over half of the average rainfall for the whole of November fell in parts of the Midlands and Yorkshire.
If you have images and/or videos of flooding or an eye witness account that you would be happy to share on an interactive flood impact map we are developing please send them [email protected]
What caused flooding in the UK in November 2019?
A large area of prolonged rainfall fell on parts of the UK in November 2019. Some areas experienced the whole of Novembers average rainfall over a period of 24 hours. Sheffield experienced 84mm of rainfall. The rainfall was caused by an area low pressure stalling over the UK.
Further reading/watching:
BBC Weather Overview
What were the effects the extreme weather in November 2019?
About 500 homes have been flooded in Doncaster with more than 1,000 properties evacuated in areas hit by the floods.
South Yorkshire Fire and Rescue said it had declared a major incident on the evening of Friday 8th November and firefighters rescued more than 40 people from the Fishlake area, near Doncaster. Residents of Fishlake said it was the first time the village had flooded in 100 years.
Empty coffins were seen floating inside the workshop of a flooded funeral parlour in the village.
Some villagers had to spend the night at a nearby pub, where staff said they had seen people crying because of the devastation.
The village church is collecting food to distribute to residents and roast dinners were delivered on Sunday to those who had remained in their homes.
Reseidents have complained that the River Don has not been dredged recently.
According to the BBC, Adrian Gill, a flood manager at the Environment Agency, said did not currently dredge the River Don “because we don’t think that’s the right thing to do” but the situation could be reviewed in the future.
Water sports enthusiast and teacher Mark Ibbotson, from Doncaster, said he, along with his 13-year-old son Logan, had rescued more than 30 people – including two babies – from a number of streets using his inflatable boat in Bentley where homes have been hit by flooding.
One of the most severely hit areas has been Bentley in Doncaster, where flooding affected many homes 12 years ago.
One resident told BBC Radio Sheffield: “The worry is our insurance policies are expensive as it is because of the 2007 floods, so now we’re all worried whether we’re going to get reinsured.”
Extensive flooding affected Rotherham , where residents were told to stay at home and not leave unless asked to do so by emergency services. Some have been taken to safety by boats.
Dozens of people were forced to spend the night in the Meadowhall shopping centre .
In Derbyshire, the River Derwent at Chatsworth reached its highest recorded level and council workers put up sandbags around Matlock and Matlock Bath, where the river was “dangerously high” .
A number of properties in Derby city centre were flooded, however, a full evacuation was not ordered as the River Derwent didn’t burst its banks to the extent emergency services believed it would.
The A52 – the main road route into Derby – was closed westbound between the city and the M1 along with a handful of smaller roads in the county.
Residents from 12 homes in Mansfield, Nottinghamshire, were unable to return home after a mudslide on Thursday led to 35 properties being evacuated .
In Nottinghamshire, residents living in mobile homes close to the River Trent in Newark were urged to move to higher ground.
On Friday, the floods claimed the life of a woman who was swept into the River Derwent at Rowsley in Derbyshire. Her body was found about two miles away in Darley Dale. She was named earlier as Derbyshire’s former High Sherriff Annie Hall .
Trains were cancelled in Yorkshire and parts of the East Midlands as rail routes were flooded.
BBC reporter Richard Cadey said some roads around Fishlake had been closed and the village was “effectively cut off because of flooding”. He said people on the ground had told him 90% of the homes there had been flooded.
The River Don, which flows through Sheffield, Rotherham and Doncaster, hit its highest recorded level at just over 6.3m (21ft), higher than it was in 2007 when it also flooded.
Sources:
England flooding: River warnings and rail delays continue
Flooding in pictures/videos
Torrential downpours flood parts of northern England – BBC
Flooding in Yorkshire – In Pictures – The Guardian
England flooding: A tour of a flooded house in Fishlake
River Derwent Flooding – Drone Video
Helicopter captures footage of flooded South Yorkshire
What were the responses to the UK floods in November 2019?
More than 100 flood warnings were put in place across England. The Environment Agency (EA) urged people to take them seriously.
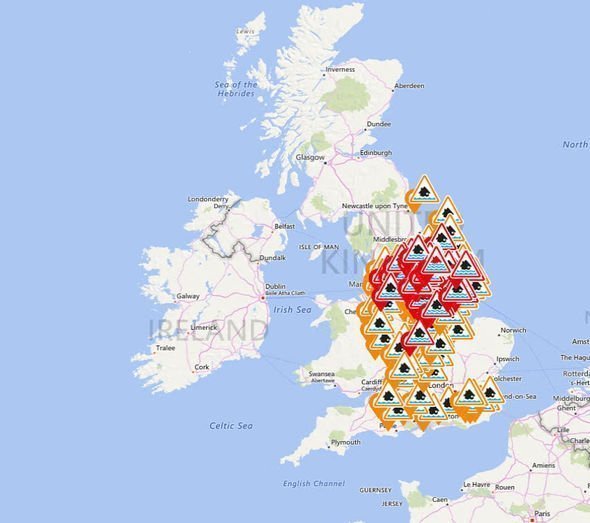
The Environment Agency took to social media to warn people about the potential impacts of flooding.
AMBER warning for flood risk today ⚠️- rain will rotate over north and north midlands bringing heavy rain on already sodden ground – take care – flood warning updates here https://t.co/K5GUW3z87V pic.twitter.com/mDDAC3uLXc — John Curtin (@johncurtinEA) November 7, 2019
The Environment Agency worked day and night to reduce the impact of flooding. The Environment Agency responded to the flood risk by working closely with police, fire and rescue, local authorities and partners to reduce the risk of flooding and keep communities safe. On the ground, Environment Agency field teams worked through the night to operate flood storage areas and pump away flood water.
A major incident was declared in South Yorkshire,
Some residents were “angry and frustrated” at Doncaster Council – claiming it had not provided sandbags early enough to prevent properties from flooding, the station reported.
Political leaders visited areas affected by floods. On the campaign trail Boris Johnson promised over £2 billion to improve flood defences.
South Yorkshire Police said it had extra officers out on patrol to “protect the evacuated areas and support those affected by the floods”.
Following a meeting of COBRA, the government’s emergency committee, Prime Minister Boris Johnson anounced the following measures :
- An extra 100 Army personnel deployed from Wednesday to support the recovery effort in South Yorkshire
- Funding for local councils where households and businesses have been affected – equivalent to £500 per eligible household
- Up to £2,500 for small and medium-sized businesses which have suffered severe impacts not covered by insurance
Six days after the heavy rain, army personnel provided support to flood-hit communities .
Environment Agency warnings
Environment Agency working day and night to reduce flood impact
How effective were the mitigation strategies introduced since the 2007 floods?
Flood defences put in place in South Yorkshire managed to significantly reduce the impact of Thursday’s floods, the Environment Agency (EA) has said.
River levels in parts of the county rose overnight to almost the same as they were in June 2007, when two people died in Millhouses and the Wicker.
Despite a major incident being declared on Thursday, the EA said the area was protected by new walls and flood gates.
The river levels around Meadowhall were high, but the EA said its defences, as well as the ones put in by Meadowhall, had lessened the damage.
Elsewhere in South Yorkshire, £3m was spent by the EA to repair and improve defences running along Ea Beck , in the villages of Toll Bar and Bentley near Doncaster.
However, people living in settlements downstream of Sheffield have complained about the impact of the recently constructed defences. In Bentley, a low-lying neighbourhood on the north side of the River Don, forlorn terraced streets are still knee-deep in water. “You don’t have to be a hydrologist to see what’s happened,” said one man interviewed by a Guardian journalist . “Sheffield built flood defences in 2015-16. They spent about £20m protecting the lower Don. So the water has nowhere to go than the next place, Rotherham and then Doncaster.” He went on to say that residents received a “red alert” on Thursday night that there was a risk of flooding. He phoned an emergency number and requested sandbags. He was told that the council was not going to distribute them because the River Don’s banks had not been breached.
When the sandbags eventually arrived the community worked together to distribute them.
South Yorkshire flooding: Defences ‘reduce impact’
Related articles:
How do you stop flooding?
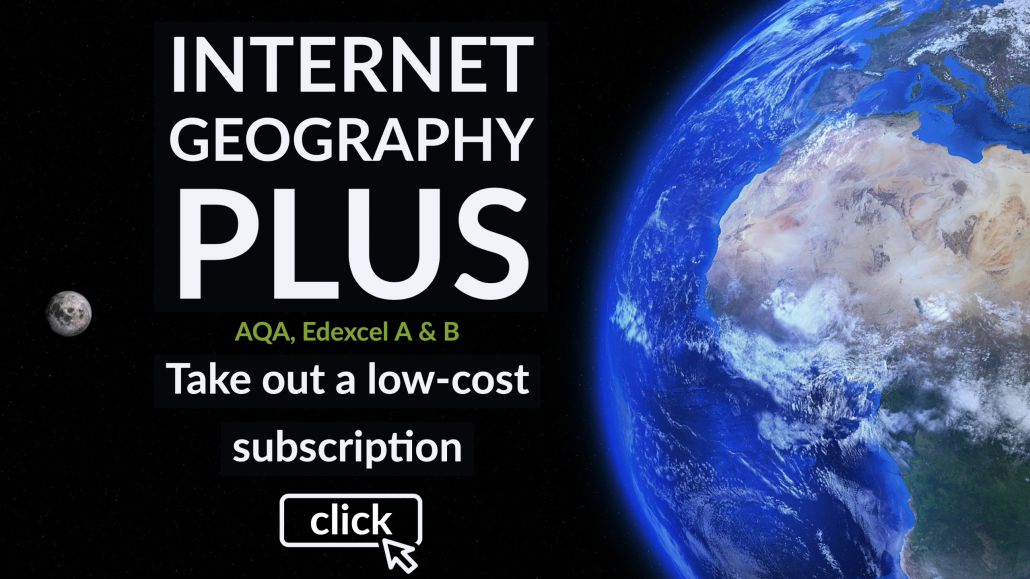
Premium Resources
Please support internet geography.
If you've found the resources on this page useful please consider making a secure donation via PayPal to support the development of the site. The site is self-funded and your support is really appreciated.
Related Topics
Use the images below to explore related GeoTopics.
Topic Home
Next topic page, share this:.
- Click to share on Twitter (Opens in new window)
- Click to share on Facebook (Opens in new window)
- Click to share on Pinterest (Opens in new window)
- Click to email a link to a friend (Opens in new window)
- Click to share on WhatsApp (Opens in new window)
- Click to print (Opens in new window)
If you've found the resources on this site useful please consider making a secure donation via PayPal to support the development of the site. The site is self-funded and your support is really appreciated.
Search Internet Geography
Top posts and pages.

Latest Blog Entries

Pin It on Pinterest
- Click to share
- Print Friendly
Advertisement
Flood risk and adaptation in Indian coastal cities: recent scenarios
- Review Article
- Open access
- Published: 04 December 2018
- Volume 9 , article number 5 , ( 2019 )
Cite this article
You have full access to this open access article
- Ravinder Dhiman 1 na1 ,
- Renjith VishnuRadhan 2 na1 ,
- T. I. Eldho 2 &
- Arun Inamdar 3
25k Accesses
77 Citations
34 Altmetric
Explore all metrics
Coastal cities contrive to spread their transformative influence both into the hinterland, along the coastline, and into the coastal waters themselves. These effects will be intensified in urban agglomerations as the concentration of population and allied activities are more pronounced there compared to the inland regions. Indian coastal cities are no exception, and it is high time to delineate these hazard-prone regions and implement proper mitigation and adaptation strategies at city scale. This review article provides an assessment regarding quantification, management and climate change impacts of flood risks in Surat, Mumbai, Chennai and Kolkata, which are the most populated coastal cities in India. The flood impacts considered in the existing or prevailing analyses are associated with adverse effects on population, land use of cities, transportation and economy caused by different types of riverine and urban flooding, though coastal flooding, tsunami and storm surge effects are less studied. Mumbai and Kolkata are relatively progressive in the assessment of flood risks and adaptation. The present article also suggests strategies to evaluate the relative progress in the assessment of past and future risks and adaptation. We also discuss the mitigation and adaptation strategies considering the historical importance of these cities. We propose that the strategies should be implemented considering public opinion and should be initialized at the grass root level. Though it is technically difficult to re-plan the city structures in the current scenario, it is possible to adapt to and mitigate the effects of natural hazards through suitable planning and management with the integrated cooperation and involvement of citizens and government as well.
Similar content being viewed by others
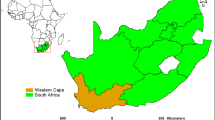
Flooding trends and their impacts on coastal communities of Western Cape Province, South Africa
Kaitano Dube, Godwell Nhamo & David Chikodzi
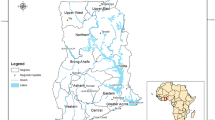
Causes, impacts and coping strategies of floods in Ghana: a systematic review
Henry Mensah & Divine Kwaku Ahadzie
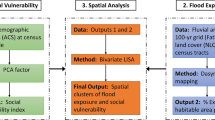
Flood exposure and social vulnerability in the United States
Eric Tate, Md Asif Rahman, … Christopher C. Sampson
Avoid common mistakes on your manuscript.
Introduction
Urban centres are dynamic interfaces and regions of intense economic activities. The growing significance of urban areas is recognized by including Goal 11 (sustainable cities and communities) in the United Nations Sustainable Developmental Goals (SGDs). Cities are knowledge and innovation hubs, that can drive the urban system towards better governance of health and the climate within cities, and are centres which catalyse societal transformations (de Oliveira and Doll 2016 ). Most of the urban regions are located in coastal areas (Small and Nicholls 2003 ). As urban areas grow, correspondingly the population and its allied pressures also increase in the coastline. In addition to natural changes, these coastal environments face multifaceted hazards due to anthropogenic fossil fuel combustion, fertilizer use, sewage input, industrial activities, tourism, overfishing, aquaculture farms and marine traffic (VishnuRadhan et al. 2014 ). The coastal cities are important drivers of socio-economic development as well as sources of environmental challenges (von Glasow et al. 2013 ), and nearly half of the world population now lives within 200 km of the coast, and it is expected to reach 75% by 2025 (UNESCO 2009 ; Creel 2003 ). Currently, India is the second most populous nation and has large coastline compared to most of the other nations around the globe; the pressure in its coastal zones is beyond imagination. India ranks 18th in terms of coastline length, and the total coastline is 7517 km including the Andaman and Nicobar Islands in the Bay of Bengal and the Lakshadweep Islands in the Arabian Sea (IYB 2007 ). The majority of the population of this agriculture-dependant country in the Indian Ocean rim is depended on the Indian summer monsoon for their survival and daily livelihood. As the monsoon is one of the most unpredictable climate phenomena in the globe (Jaiswal et al. 2018 ; Mahmood et al. 2018 ; Shahi et al. 2018 ), it can substantially affect the population not only in terms of their basic livelihood, but also by inducing geohazards such as flash floods and inundation. In the case of coastal cities, the effect will be exacerbated as these are densely populated and are prone to sea-level rise, storm surges and tropical cyclones, of which recent studies portraits are intense under climate change scenarios (Mendelsohn et al. 2012 ; Rahmstorf 2017 ; Vitousek et al. 2017 ).
The city scale has ever been more recognized for risks management and adaptation in recent years (Bai et al. 2018 ; Ürge-Vorsatz et al. 2018 ; Rodriguez et al. 2018 ; Solecki et al. 2018 ). Analysis within the city boundary is more relevant for local administrations such that decisions for adaptation can be facilitated at an applicable level of governance. There are a number of significant impacts on cities which are directly related to climate change such as surface sealing by concrete (urban heat islands) and urban floods (Lindley et al. 2006 ). The developing countries are having greater vulnerability than developed countries at city scale, because of rapid increase in population as compared to the capacity of infrastructure, resulting in the deficit of their adaptation measures towards climate variability and future exposure to flood risks (Hunt and Watkiss 2011 ). The definition of the coastal megacity is debatable in the literature because of the inconsistency in the threshold of megacity which is 1, 8 and 10 million people (Cross 2001 ), but the United Nations has defined the megacity as having population more than 10 million (UN 2008 ). We have considered coastal megacities following Nicholls and Small ( 2002 ). Kolkata is located 145 km away from the coastline, but is considered as a coastal megacity because of the direct effect of marine processes and deltaic settings (Sekovski et al. 2012 ). Though Surat is not a megacity, the city is growing in terms of population and infrastructure and can be a potential future megacity.
This review article presents evidence-oriented flood risks and adaptation strategies in select coastal cities in India based on published literature, in addition to reviewing the methodology of information utilization. We also delineate the potential impacts of flood-induced risks in the select coastal cities: Surat, Mumbai, Kolkata and Chennai. According to the previous studies (Revi 2008 ; Bhat et al. 2013 ; Ranger et al. 2011 ; Joerin et al. 2012 ; Nicholls et al. 2008 ; Dasgupta et al. 2013 ), the most important risks posed by floods in Indian coastal cities are primarily due to (1) the riverine flooding and extreme precipitation, (2) the effect of cyclonic storms, tsunamis and tidal inundation, (3) failure in urban planning and unsustainable land-use practices. Flood risks are usually coupled with cyclones, storm surges, tides and tsunami across different geographical locations having a difference in impact categories, including riverine flooding, urban flooding, population growth, transportation, energy demand and climate variability. Füssel and Klein ( 2006 ) have mentioned the importance of qualitative approaches over quantitative estimation during the assessment of large future risks to minimize the uncertainty. The literature mentioned in this review is supposed to be indicative only of the true scope and extent of flood risks and climate change impacts along the major Indian coastal cities.
This study integrates the existing scientific studies about floods (impacts, methods and adaptation) in major Indian coastal cities precisely to make it easy for other researchers who want to pursue advancements in the similar domain. This work considers the assessment of different methodologies also, particularly techniques adapted in different studies, which results in lack of consistency. Consequently, several studies have highlighted different methods, including the action of climate models, socio-economic scenario, index-based approach, GIS and the monetary valuation of market/non-market impacts. Comparability among different approaches may assist more easy transfer of useful discoveries and observations between cities. The existing coastal city studies show that sectoral stakeholder engagement is the key to maximize the benefits of adaptation activities. We are highlighting the need of future experiments relevant to overall objectives and necessary actions of flood impacts coupled with climate change scenario. The goal of this review article is to evaluate the causes, potential risks and measures to control them at city scale under the pressure experienced along the dynamic coastline of India and is intended towards the assessment of flood impact considering all possible scenarios. Moreover, there are no review articles exclusively dedicated in addressing the flood impacts and adaptation considering the Indian coastal urban regions.
In this study, four maximum populated coastal cities along the Indian coastline, two at the west (Surat and Mumbai) coast and two at the east (Chennai and Kolkata) coast, are chosen. The selection of cities from both the west and east coasts is due to the fact that the climatic/oceanographic conditions along the two coasts are different (Fig. 1 ). These cities are vulnerable to floods by multiple causes such as rivers in the city itself (adjacent or central), faulty urban design and planning, the dynamic and vulnerable coastline, flash floods, storm surges, cyclones and tsunamis. Table 1 indicates the causative factors leading to the occurrence of floods in these coastal cities.
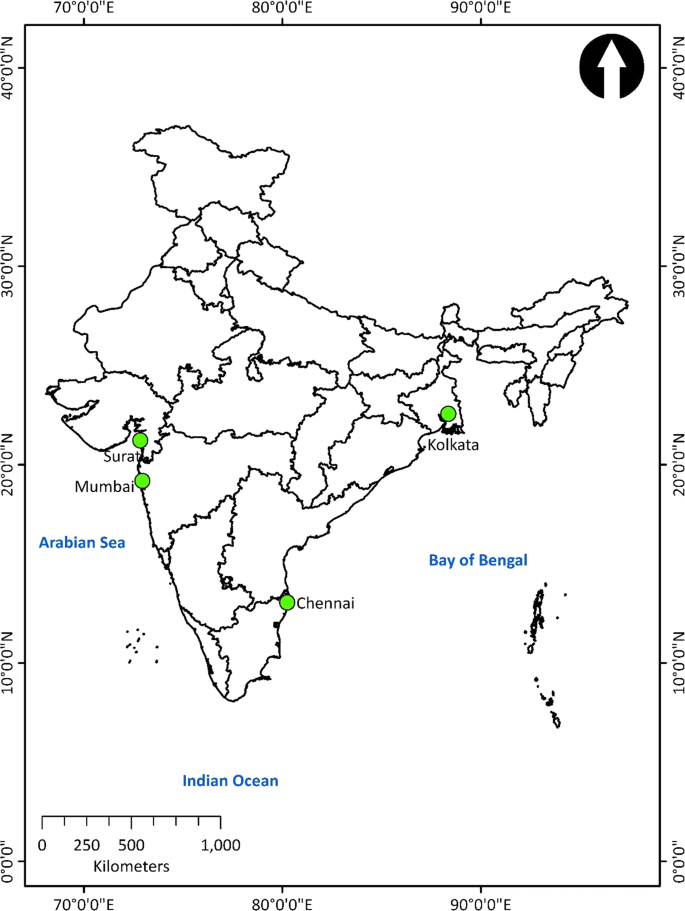
Locations map of study areas
Surat is a historical coastal city located on the bank of the Tapi River, Gujarat state. The Surat port was an active hub of trade and commerce in the past, and the city reached its utmost prosperity during the sixteenth and seventeenth century. Surat was also the main port of the Mughal Empire (Gupta 1987 ). The British East India Company established one of its first Indian factories at Surat in 1612, and this English factory has been called the cornerstone of the British Empire in India (Rawlinson 1920 ). The colonial past of the city resulted in providing well-documented past flooding events in the city. Imperial Gazetteers and written historical accounts documented details of the past floods prior to the instrumental records (Kale et al. 1996 ). The construction of an inner wall in the city was commenced by the British in the year 1664 as a flood protection structure with gates that were closed in the event of a flood, and the construction of the entire wall was completed in the year 1707. The city area expanded from 8.12 km 2 (prior to 1961) to 326.5 km 2 in 2009 (SMC 2011 ). The city can be divided geomorphologically into a coastal zone and an alluvial area. The coastal zone is composed of marshy shoreline with an extensive tidal flat stretch intercepted by estuaries. The alluvial area is marked by alluvial deposits from the Tapi River (Patel et al. 2017 ). Flooding occurs in the city when there is a sudden release of water from the Ukai dam (constructed in 1972) which is located 100 km away from the city. Floods can also be caused by seawater intrusion through different creeks which are also known as Khadi floods, less devastating than the Ukai floods though it significantly affects the vulnerable households along these creeks (Bahinipati et al. 2015 ). The city population has grown tenfold since 1951, and the municipal area has increased from 8.1 km 2 in 1951 to 327 km 2 recently (Bhat et al. 2013 ). The population was 5.9 million in 2016 and is projected to be 8.6 million in 2030 (UNDESA 2016 ), which shows more vulnerability in the near future.
Mumbai, the most populous Indian city, located along the western coast of India, started trading activity during 1675. It is the largest port in western India and simultaneously known as the commercial and financial capital of India (Pacione 2006 ). The megacity currently ranks as the 5th largest city (in terms of the population) in the globe (UNDESA 2016 ) and is originally composed of seven islets. Anthropogenic reclamation primarily caused the islets to merge and form the current Mumbai city. It is encircled by the Arabian Sea to the west and harbours the Thane Creek inlet to the east. Mithi River is located in the centre of the city having origin from Vihar and Powai Lake. Weather is typical humid and coastal sultry, and annual rainfall ranges from 15 to 20 cm (Vaz 2014 ). The population of the city during the first census of India in 1872 was around 65,000 (Da Cunha and Jose 1902 ). Since then, the city has developed extensively over the years, and correspondingly the population increased to around 21.3 million in 2016 (UNDESA 2016 ), which is projected to pass 27 million in 2030. This has created enormous pressure on the land and resulted in substantial land-use changes in the region. Burgeoning population and encroachment have resulted in the unavailability of land for residential and commercial purposes, leaving the city with the only vertical growth option. This space crisis may have prompted the state government to raise the floor space index (FSI) in 2018, which will further develop the city vertically. The city experiences frequent spells of flood during monsoon time. The primary reason for the flooding stems from the fact that the shape of the present Mumbai city was moulded by the reclamation of water-logged areas between the original seven separate islands. The reclaimed space between the islands lies below the high water level. Whenever the rainfall persists for a longer time (7–10 h) and it coincides with the time of high tides, water cannot recede into the ocean and compounds the flooding (Gupta 2009 ). Apart from this, other important reasons for flooding are mainly anthropogenic. These include inappropriate levels of outfalls, the increase in the run-off coefficient due to the urban landscape, the loss of holding ponds due to land development and encroachments on drains and obstructions caused by utility lines being crossed (MCGM 2014 ). The yearly flooding in Mumbai incurs huge economic losses due to the economical–social disorientation and associated shutdown, ultimately affecting the nation’s economy. small- and medium-sized enterprises (SMEs) often bear the cost of flooding as the industrial estates located near chronic flooding spots experience recurrent flooding at least two or three times every year (Schaer et al. 2018 ). Figure 2 shows the estimated total losses for a 1-in-100-year flood event in Mumbai under five scenarios.
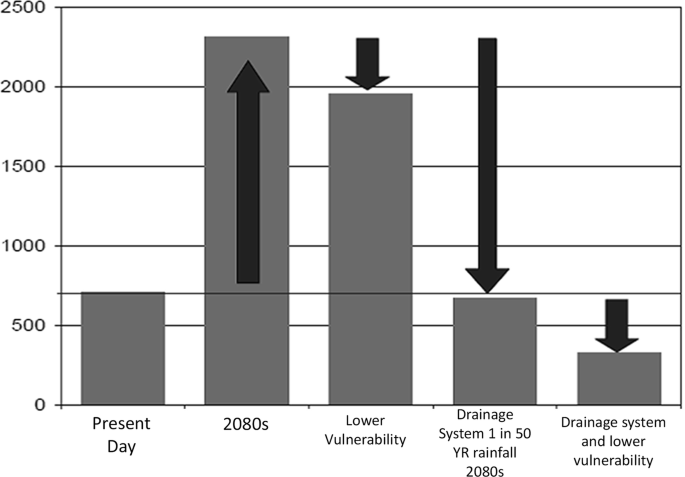
Estimated total losses for a 1-in-100-year flood events in Mumbai under five scenarios (following Hallegatte 2010 )
Chennai, formerly known as Madras, was formed in 1600 and is the capital city of Tamil Nadu state. The city is located at the southern east coast of India, bounded by the Bay of Bengal in the east. The region has persistent as well as eventful archaeological and colonial history. The City is drained by three major rivers, namely Adyar (to the south), Cooum (through the centre) and Kosasthalaiyar (to the north). The city faces a number of risks which include climate-related as well as human-induced reasons (Gupta and Nair 2010 ). Chennai receives an average approximately 1300 mm of rainfall per year, but the majority of this (~ 800 mm) falls during the north-east (NE) monsoon months (October–December). The population of the city was 777,481 (1941 Census of India) before the independence of the country, which drastically increased thereafter to a current population of 10.16 million in 2016 (UNDESA 2016 ). This number is projected to continue increasing to 13.29 million in 2030. The rise in population leads to intensive developmental activities and land-use changes. The region has plain terrain, thus lacking a natural gradient for free run-off. In order to wade off a potential flood, the city requires natural buffering mechanisms such as wetlands, marshes and open lakes. These buffering factors are significantly affected by the construction and developmental activities allied to the city growth as well as encroachments. For example, the alarming decreases in the area of the Pallikaranai marshland from 5000 ha in 1970 to 988 ha in 2008 (Stephen 2016 ). Recent devastation by the flood waters in the region was wrought mainly by the shrinkage of wetlands and marshes. Major impacts of flooding on the booming IT industry in the region and sustainability of housing sector can be expected in the years to come (Suriya et al. 2012 ).
Kolkata, previously known as Calcutta, is the capital of the Indian state of West Bengal. Calcutta was the last of the port cities to be founded by the English, and the suburbanization had been started by the British during the last decades of the eighteenth century (Kosambi and Brush 1988 ). The modern city of Kolkata was developed on land recovered from the wetlands following the Dutch model of cities built along the Rhine River despite geographical challenge similar to those in Kolkata (Rumbach 2014 ). The city is often mentioned as the city in the swamps (Murphey 1964 ) and has been found to be one of the world’s most flood-prone coastal cities (Balica et al. 2012 ). Though situated away from the Bay of Bengal, the city is considered as a coastal city because of the deltaic setting. This megacity has a tropical climate and is subjected to extreme precipitation during monsoons with an annual rainfall of around 160 cm. It is situated on the bank of the Hooghly River and has an area of 1851 km 2 . The region is prone to flooding primarily due to the flat terrain and the drainage outfalls which are tide-dominated. Flooding occurs during excess rainfall on the catchment as well as during high-tidal conditions. The drainage sewer system in the city was designed during the British era and is not capable of handling the current run-off scenario. The sewer system also suffers from siltation and a consequent reduction in conveyance capacities irrespective of the efforts by the Kolkata Municipal Corporation (KMC) in renovating the drainage pipelines (Sen 2013 ). The population increase (14.98 million in 2016) (UNDESA 2016 ), rapid urbanization and the corresponding land-use changes have substantially reduced the flood-resilient efficacy of the region in recent years.
Research methods and comparative assessment
This section briefly provides insights into major flood events in the study regions and the different methodologies adopted in various studies for elucidating flood-induced risk in the above-mentioned coastal cities (Table 2 ).
Flooding is common in the region which depends on climate, seasonality and other local factors. Five major flood events were reported since 1979, and the flood event of 2006 was devastating. Major flood events are reportedly caused due to monsoon-induced rainfall events (the peak flood is observed during August) (Agnihotri and Patel 2008 ) as well as by the emergency opening of the Ukai dam. The various factors which contribute to the flooding of Tapi River are the increase in population and allied factors such as built-up area increase, silting up of riverbed and embankment construction. Though the Ukai dam in the region was constructed with an additional aim to prevent floods, major floods during years 1998, 2004 and 2006 occurred due to the emergency opening of the dam when its capacity is overthrown (Joshi et al. 2012 ). The Khadi floods also pose a flooding threat (e.g. 2004, 2005 and 2007) in addition to the flooding of the Tapi River. Surat was selected as one of the pilot cities in the Asian cities climate resilience network in 2008. The major flood events also increase the risk of vector-borne disease in the region (Bhat et al. 2013 ). Jariwala and Samtani ( 2012 ) detailed the natural and anthropogenic cause of the flood in the southern part of Surat city, where the storm water is discharged through Mindhola River and its tributaries. Some of the anthropogenic factors listed are land reclamation on the bank of creeks, construction of bridges and suspended solids/non-degradable substances in city effluents. Natural reasons include precipitation pattern, catchment topography, bed gradients and the cross-sectional area available for flow. The peak flood is estimated from hydrological and catchment data using rational formula, and accordingly tributary cross sections were designed to contain the estimated peak flood. Jariwala and Samtani ( 2012 ) proposed the modification in the channel section of the most vulnerable stretches (Mithi and Kankara), so that it can contain peak flood waters. Joshi et al. ( 2012 ) had done urban flood mapping using geospatial techniques at Surat city. They used the Geo-Coded Indian Remote Sensing (IRS-1D) satellite image of April 2005 and Topo-Sheets at 1:50,000 scales collected from office of Survey of India and generated flood inundation map for the West Zone area of Surat City for different flood water levels. It was found that the inundation will start at water level above 4.0 m in the low-lying area in the West Zone area of Surat. The study also showed that the 95.38% area will be inundated when water level will rise at 12.0-m level.
A two-dimensional hydrodynamic model was used to simulate flooding (Ramirez and Rajasekar 2015 ). The methodology includes three stages: (1) simulation of Ukai dam release discharge reaching the city, (2) simulation of the combined effect of the tides and the dam released water, (3) delineating the vulnerable infrastructure and population. A loss and damage assessment of Surat City due to floods was carried out (Bahinipati et al. 2015 ). This study exclusively considered the textile industry which was affected during the 2006 floods. The storm drainage from the Surat city passes through various tributaries (creeks) and ultimately drains into the Mindhola River. Following the floods during 2006, flood hazards mapping of the urban floodplain of the Tapi catchment area was carried out using GIS and remote sensing techniques (Singh and Sharma 2009 ). Ukai dam release scenarios combined with tidal effects were used to run a two-dimensional flood model (CAESAR-Lisflood) using a fine spatial resolution (30 m) topography (Ramirez et al. 2016 ). Simulations were carried out for extreme conditions, and probabilistic flood risk maps were prepared for the city. The flood impact assessment and the extent of inundation in the low-lying area are carried out (Patel et al. 2017 ) using a 1D/2D coupled hydrodynamic model (HEC-RAS 5) considering two hundred and ninety-nine cross sections, five major bridges and two hydraulic structures along the river course. The model set-up considered the conditions (tidal level at the river mouth and the Ukai Dam release) during the 2006 flood as boundary conditions. The results indicated that ~ 75–77% area of the city was inundated during the flood. Areas within the city which are prone to flooding and submergence were identified using Digital Elevation Model and Geographical Information System (Patel and Dholakia 2010 ). Modelling studies incorporating climate factors are almost absent in the region. As the city is predicted to be prone to flooding induced by climate change impacts, there is a need for studies addressing this issue.
Mumbai city
The Mumbai region is prone to severe floods annually, and the flood of 2005 (Gupta 2007 ) was the worst in near history. The region is flood prone primarily due to its geography, which was shaped both by natural and by anthropogenic activities. The heavy summer monsoon rainfall together with the anthropogenic geographical alterations aggravates the flood risk. The land is heavily reclaimed and most of it is below the high-tide line, which can cause sea water entering to the complex drainage system of the city, resulting in mass inundation, as occurred during the 2005 floods. Urbanization is the most important driver of flood risk in the city. The inadequate drainage system and an ineffective spatial planning increase the flood risk in the region. There had been a surge in the number of studies regarding flood in the region following 2005 flood event. Major flood events in Mumbai are studied and compiled by Hallegatte et al. ( 2010 ). They used risk quantification following a standard catastrophe risk modelling framework combining estimates of hazard, exposure and vulnerability, which can give an estimate of economic damage and exposure of population to flood risks in various probabilities. Additional damages from the floods are also included in the framework. Simulation using climate model and further downscaling indicate high potential sensitivity of flood risk to climate change (Ranger et al. 2011 ). An urban flood model, Storm Water Management Model (SWMM), was used in the study. The land use/land cover (LULC) of the region is derived by analysing observations from the IRS LISS III satellite. Chatterjee ( 2010 ) discussed the response of the informal settlers in 2005 flood events in Mumbai. The study showed that informal settlers have to employ a combination of structural means and complex networks of assistance to recover from flood-induced risks. Ramesh et al. ( 2008 ) used extreme value analysis (EVA) to find out the hourly maximum rainfall, which is helpful in the estimation of flood levels during maximum rainfall conditions. The changes in the urban pattern (1973 and 2004) of the city were mapped using Landsat MSS and ETM+ data (Pavri 2010 ). A land-use map, using an unsupervised classification, and an elevation model using Shuttle Radar Topography Mission (SRTM) data were prepared, to identify the zones vulnerable to floods. A FEM-based flood simulation model was developed to simulate rainfall events consisting of flooding as well as non-flooding events (Shahapure et al. 2011 ). The model was integrated with the GIS and remote sensing database and could simulate the flooding event and the required detention pond. A Participatory Spatial Risks Mapping was carried out in the Dharavi region which encouraged the community participation which includes town watching and hazard mapping (Samaddar et al. 2011 ). The method was proved to be a very promising and empowering tool for local communities in tackling flood issues. An artificial neural network (ANN)-based flood management system was developed (Subramanian 2012 ), which helps users in data processing and can serve as an effective real-time flood tackling decision management system in the region due to its flexible and interactive nature. A methodology for rapid inundation mapping was developed for a data-sparse condition considering two scenarios (Gupta and Nikam 2013 ). The scenarios include continuous rainfall at 50 mm/h (the return period of 2 in 1 year) and 100 mm/h (the return period of 1 in 1 year), each for 1 h. This helped in delineating areas prone to flood water inundation due to these intensities. The flood simulation in the coastal urban region was carried out using an integrated flood assessment model in which the spatial data sets were organized by a web-based GIS framework (Kulkarni et al. 2014 ). The model could simulate the flood event during the extreme rainfall event of 2005. The spatial and temporal variation of LULC change for the coastal urban catchment area of the Mithi River was evaluated using toposheets and remote sensing data (Zope et al. 2015 ). Flood hydrographs were formulated for various conditions of LULC change and floodplain maps as well as flood hazard maps were derived. The models HEC-HMS and HEC-RAS were integrated with HEC-GeoHMS and HEC-GeoRAS for the preparation of maps. The study contends that major flooding can occur even in marginal flow conditions in the catchment when there is tidal influence. Another LULC change analysis was carried out for the Oshiwara River catchment in the region (Zope et al. 2016 ) to understand the impact of LULC change and urbanization on floods. The study found out an increase in the flood inundation area in the region.
Kolkata city
Kolkata city is prone to annual flooding during monsoon time and during extreme events such as cyclones. The city drainage network is too old, and the existing network is not sufficient for the entire city. The parts of the city were once wet lands, and the slope of the region as well as the wet land nature is posing threats to the drainage. A high-intensity rainfall event combined with high-tidal condition will always result in water-logging condition. Heavy siltation and inadequate maintenance of the sewer system have reduced the hydraulic capacity, which is also increasing the flood risks. The region is undergoing land subsidence (Chatterjee 2010 ), and this can be a boosting mechanism for future floods in the region. Presently, the population in the region has adapted to flood risks as it is not very severe and long lasting. Present adaptation strategies can change the wake of severe flood induced by climate change scenarios. There are three factors responsible for flood risks in the area: (1) natural factors, (2) developmental factor and (3) climate change factors. The natural factors include the low relief and topography, natural subsidence together with cyclones, storm surges and heavy rainfall. Unplanned urbanization, the low capacity drainage system, siltation in sewerage channels and human-induced subsidence are included in developmental factors (Dasgupta et al. 2013 ). The Kolkata Municipal Corporation (KMC) has recently introduced (September 2018) a comprehensive city-level Flood Forecasting and Early Warning System (FFEWS) making Kolkata as the first city in India having this kind of facility. This will empower the city administration and citizens in efficiently manage flood risks and damage.
An integrated management of storm water management in the region was discussed by Bose ( 2008 ). Remote Analysis of Vulnerability by Non-linearization Algorithm (RAVANA) was used to identify the extent of flood inundation in the Kolkata city (Chakraborty 2010 ). An array of automatic rain gauges and digital water-level sensors were used to monitor the rainfall distribution and water levels in the drainage channels in real time (Sen 2013 ). A framework for a real-time flood inundation forecasting model was proposed using a sewer flow–overland flow combined model, the results of which can be disseminated to the public through the internet. The flood vulnerability of the city was assessed considering intense precipitation events for return periods of 30, 50 and 100 years using rainfall data, emission scenarios and the sea-level rise for 2050 (Dasgupta et al. 2013 ). The outcome was a geographically overlaid, location-specific inundation depth and duration forecast. The study also projected the relevance of de-silting the sewers in reducing the vulnerability. The vulnerability of informal settlements in Kolkata to the flood risks indicates the dependence towards various socio-economic and infrastructure variables (Rumbach and Shirgaokar 2017 ). The study proposed that the disaster reduction policies should focus on lowest income groups. An open-source, coupled flood model having a surface model which uses a simplified numerical scheme and SWMM drainage network model was developed to study historical flooding in Kolkata (Courty 2018 ). The developed model has been proved to be a useful tool in flood risk mapping and drainage network design.
Chennai city
Chennai coastal region is prone to Tsunamis (e.g. in 2004) and cyclones; both the factors can cause coastal flooding. The flood in the region is manifested mainly by anthropogenic reasons (Drescher et al. 2007 ). Chennai lacks a natural gradient for free run-off; thus, an effective drainage system is very essential. The present drainage system is not sufficient for the growing city. The Cooum and Adyar rivers in the Chennai city also play a major role in the floods. The river banks are also heavily encroached. Chennai witnessed several catastrophic floods in recent years associated with heavy rainfall events and cyclonic depressions, which often lead to flooding of major rivers and clogging of the drainage system. A major flood event occurred in December 2015 and was reported as one of the most disastrous floods in the history of the region. The flood event affected 2 million people and caused the loss of properties worth US$ 80,000 million (Ramasamy et al. 2018 ). A surge in studies addressing the causes, effects and impacts of floods in the region was observed following the devastating flood of 2015.
Geocommunication and geovisualization were used as interactive participatory mapping tools to understand the risk due to extreme precipitation in the coastal areas of Chennai (Glaser et al. 2008 ), and the results were publically accessible through an information portal. Strategies to reduce flood risks were detailed in Gupta and Nair ( 2010 , 2011 ), which include directions such as the protection of lakes and open water bodies from encroachment and eviction of existing encroachments by governmental agencies. Flood avoidance, flood tolerance and flood resilience strategies should be combined into an integrated mode to build up flood resistance in the city. A basin model was developed by using a hydrological model based on remote sensing (HEC-GeoHMS), and the run-off due to precipitation was simulated using the HEC-HMS model in the Thirusoolam sub-watershed in Chennai (Suriya and Mudgal 2012 ). Flood hazard and flood zone mapping were also carried out, and the study showed the impacts of urbanization on hydrological processes. Different available methods to tackle the damage caused by the flood and the methodology to assess the economic loss in the Velachery region, Chennai, were discussed by Suriya et al. ( 2012 ). The methods include problem identification tasks composed of focus group discussion, stakeholder meeting, the questionnaire survey, succeeded by the data set preparation and flood damage assessment. Soft systems methodology (Rich Picture Diagrams and CATWOE analysis) was used for an integrated flood management and assessment in Adyar watershed in Chennai (Suriya and Mudgal 2012 ). A vulnerability assessment study was carried out in the region using satellite data and GIS techniques. Shoreline change rates were derived, and the Chennai coastal region was classified as a highly vulnerable zone (Mariappan and Devi 2012 ). Pairwise ranking (ranking and prioritizing a decision criteria) and force field analysis (visual identification and analysis of the forces affecting the flood situation), which are participatory action research tools used, were also used. Hydrological (HEC-HMS) and Hydraulic (HEC-RAS) modelling was used in feasibility analysis to control the flooding (Gokilashree 2015 ). The study proposed a new drainage channel in south-west Chennai, which will reduce the inundation area by 70%. Some flood mitigation and prevention strategies such as the revival of Buckingham Canal and renovating the surface water bodies in the region were proposed (Selvaraj et al. 2016 ), in addition to the call for better strategies considering future extreme scenarios. Jameson and Baud 2016 showed the influence of a variety of knowledge about the flooding in creating a more efficient flood management system in Chennai. Meteosat IR rainfall data, measured using a multispectral rainfall signature-based rainfall estimation technique, were used to monitor the 2015 flood (Mishra 2016 ), and the 2015 flood scenario was analysed using an integrated geographical information system (GIS) and multicriteria decision analysis (MCDA) method (Seenirajan et al. 2017 ). Ahmed and Kranthi ( 2018 ) found that around 18% of the Chennai Metropolitan Area was affected by the 2015 flood event, using satellite data from Landsat-8 OLI, Sentinel-1 and CartoDEM-3 R1. Arabindoo ( 2016 ) discussed the argument among environmentalists and the administration where the former targeted the haphazard urbanization as a strong reason for the floods in the city and the later considered it as an unprecedented climate anomaly in the history of the city. Sharma et al. ( 2017 ) showed the potential of global rainfall products (Tropical Rainfall Measuring Mission (TRMM)) and global forecast precipitation products (Global Ensemble Forecast System (GEFS) in successful flood mapping of the region. Methods such as map and site analysis, focus group interview and questionnaire survey were employed by Muneerudeen ( 2017 ) to compose urban and landscape design strategies for flood resilience.
Climate change impacts and flood risks
There is a growing scientific consensus that the climate is changing (Hartter et al. 2018 ), and the impacts on the coastal zone can be in the form of sea-level rise, the increase in the frequency of extreme wind events, the change of the annual frequency of winds, the increase in storm surge events frequency, higher waves, changes in wave direction and changes in coastal currents (Karambas 2015 ). These impacts can result in urban flooding events of varying intensities ultimately affecting the prevailing city metabolism. The impacts of the changing climate are exacerbated in urban areas due to the immense anthropogenic activities centred around these regions. All the cities addressed in this study have been through recent flood problems related to the changing climate. There are less studies addressing the climate change impacts on the flood events in these urban areas, and there is a knowledge gap regarding these realms.
For Surat city, various climatic factors, such as precipitation, temperature and sea level, were identified in the region which increase the flood risks and can induce coastal inundation. Climate models also indicate higher future precipitation in the region and an increase in number of rainy days. The models also predict the increase in minimum and maximum temperature of the region with a monthly average maximum temperature increase by 0.5 °C (Bhat et al. 2013 ). A Climate Change Impact Assessment using HEC-RAS model was carried out to picturize the inundation scenario in Surat (Parikh et al. 2017 ). A hypothetical situation was considered (a 50% increase in water inflow in the Tapi River) as a possible impact of climate change. The results indicated that there will be more inundated areas in the region as a result of climate change impacts.
For the city of Mumbai, previous studies indicate that the region is likely to be highly vulnerable to climate change and that the flood-prone and reclaimed land is occupied by the majority of Mumbai population (Patankar et al. 2010 ). The city is vulnerable and exposed to climate change-induced hazards stemming from sea-level rise, heavy precipitation, storm surge and tropical cyclone risks (Hallegatte et al. 2010 ). An assessment of climate change impacts in the region observed that by 2080 the likelihood of floods similar to the 2005 flood is more than double (Ranger et al. 2011 ). The climate change-induced floods can also inflict current and future economic losses in the region (Kumar et al. 2008 ), and the cost was found to be enormous. The single-value water surface method was used to find out the probable flooding for the various sea-level rise scenarios, and it is observed that the rate of coastal inundation is directly correlated with the sea-level rise (Singh and Kambekar 2017 ). Climate change-induced sea-level rise scenarios were used to study (Pramanik 2017 ) the impacts caused by these scenarios on the Mumbai coastal region and concluded that the sea-level rise can potentially reduce the drainage gradients in the city and can induce flooding conditions.
For Kolkata city, climate change factors responsible for flooding in the region comprises the sea-level rise, extreme precipitation and storm surge. Increased flooding in the region is caused by increased precipitation together with high tide and extreme storm surge. Dasgupta et al. ( 2013 ) studied the hydrological and hydraulic impacts of extreme precipitation due to climate change by 2050. The study employed three models to capture the flooding: (1) Soil and Water Assessment Tool (SWAT) (hydrological model), (2) Hydrologic Engineering Centre-River Analysis System (HEC-RAS) (hydraulic model) and (3) Storm Water Management Model (SWMM) (urban storm model), and historical rainfall data from continuous recording rain gauges for 25 years. The study urges the need for considering the climate change effects in all the future adaptation strategies for the Kolkata city. The heavy rainfall and storm surge combined with the high-tide result is heavy flooding and is a major problem for the river-side dwellers of the city (Ghosh 2015 ), which will be exacerbated in this era of climate change, and a world bank study modelled the impact of climate change on increased flooding in the region (World Bank 2011 ).
For Chennai city, there is a knowledge gap in the climate change assessment of the Chennai city since there are no major assessment studies till date. It is high time to address climate change impacts on flood risks and associated variability in the wake of present regional as well as global climate scenarios. van Oldenborgh et al. ( 2016 ) ruled out the possibility of the effect of global warming during the extreme rainfall event (caused by substantial flooding and inundation) of December 2015. This was attributed to the counteracting effect of aerosols and greenhouse gases. Some of the recent case studies about Chennai floods of December 2015 investigated the impact of climate change based on extreme rainfall data and the relationship with the global phenomenon like El-Nino and La-Nina, but the observations are contrary because of different data sets and methodologies for analysis (Krishnamurthy et al. 2018 ; Boyaj et al. 2018 ).
Flood risk and impacts on the cultural sites and monuments
The flood risks and impacts on the heritage sites and monuments in the coastal cities are a less addressed issue globally. A large number of cultural world heritage sites are concentrated near the coasts (Marzeion and Levermann 2008 ). Currently, there are lot of instances regarding disasters affecting the cultural heritage and monuments, but this realization is still not widespread (Taboroff 2003 ). The cultural heritage and monuments in historical urban areas have not received attention or support they warrant (Jigyasu 2016 ). The integration of cultural heritage and development in urban areas can result in a substantial increase in the sustainability and diversity of proposed development. This can also provide opportunities for employment and community participation ensuing social cohesion and peace (ICOMOS 2015 ). A holistic approach should be undertaken for the flood risk assessment in regions with cultural heritage structures, which must integrate physical, economic, social, and cultural vulnerabilities, along with perceived risk (Vojinovic et al. 2016 ). Cultural sites, historical infrastructure, monuments and objects of art can be affected by a wide variety of flood-associated impacts (Drdácký 2010 ). These include horizontal static pressure of the rising water, upward hydrostatic pressure, floating and displacement of objects due to the low-velocity stream movement, dynamic high-velocity stream action due to high-pressure water emerging through tiny openings, dynamic impacts of floating materials, the dynamic wave impacts, changes in soil properties and condition, saturation of objects and materials with water as a result of complete immersion in water, chemical and biological pollutants affecting historical objects and art works, barrier formation by the floating objects due to the combined uplift forces and flow, and post-flood effects such as change in internal climate resulting from high humidity, and appearance of cracks, the loss of cohesion, and volumetric changes of structures and materials due to the post-flood drying. There are several occasions of destruction of the archaeological sites and monuments due to flood effects even in the countries which devised substantially well and practical adaptation strategies to cope up with the flood damage inflicted to cultural sites and monuments. All the four cities addressed in this work are historical cities and have a significant place in global history. Currently, there is no authenticated database showing the extent of damage to the cultural sites and monuments in these cities due to past floods. Due to the absence of any ample legal framework, the vulnerability of the historical urban areas has been exacerbated during the disaster as well as the emergency period and post-disaster recovery phases (Jigyasu 2016 ). The recently released National Disaster Management Guidelines for Cultural Heritage Sites and Precincts (NDMA 2017 ) contain sufficient details pertaining to the directions for various departments, ministries and other stakeholders involved in the management of Cultural Heritage Sites and Precincts. These guidelines, if followed properly and systematically, can be very useful for the historical urban areas in India where there is recurring floods and associated impacts.
Adaptation framework for managing flood risks
Adaptation to extreme events such as coastal floods is very crucial in the present era due to the multi-facet nature of the causes and effects, which will be exacerbated due to the increasing population and climate change. The ever-changing and cross-boundary nature of flood risk (Jongman 2018 ) render adaptation strategies as very crucial in this era of fluctuating social and environmental conditions. Flood adaptation strategies are often intended to minimize impacts on various sectors such as built environment, human health, water quality and transport infrastructures (Wilby and Keenan 2012 ). The efficient and effective adaptation strategies should have the services of structures and facilities to defend flood, use solutions which are nature-based, have early warning systems, include financing schemes to tackle and reduce the economic loss due to the flood risk, practice risk-informed land planning (Jongman 2018 ). The regions located mainly in the tropics will experience the largest increase in the flooding frequency in the near future (Vitousek et al. 2017 ). Human adaptation to the environment is one of the most important processes of survival (Rossnerova et al. 2017 ) in the era of extreme floods. Adaptation measures in a developing country like India can bring in immediate community benefits (Mathew et al. 2012 ) which can result in the reduction in future flood impacts. Though there is a considerable improvement in the flood adaptation measures in India, the social and economic costs due to floods continue to increase yearly. Adaptation measures can play a pivotal role in managing flood risks in the near future in the four coastal cities addressed in this study, considering the huge population and infrastructure which are vulnerable to extreme flood conditions.
Agnihotri and Patel ( 2008 ) elucidated flood reduction plans for the Surat city by suggesting some preventive and curative measures by highlighting the need for flood water detention ponds and diversion of flood waters to other rivers. They used Kennedy’s theory for the design of the interlinking canals. A channel modification strategy for the Tapi River using HEC-RAS and Arc GIS software HECGeo was put forwarded (Agnihotri and Patel 2011 ) as a curative flood control measure, useful in the preparation of mitigation and adaptations plans. Bhat et al. ( 2013 ) addressed various strategies to aid Surat city to cope up with and adapt to flood risks. Flood adaptation uses built environment such as by raising the plinth height and urging to use the ground floor for parking purposes only. Low-income groups adopt their own methods to store valuables in the case of extreme events, for example keeping things in plastic pouches. Warnings using the help of information technology are also included in adaptation strategies. The megaphone warning mostly helps the low-income group during a possible flood event. There are plans to implement GIS-assisted warning system by geotagging residential complexes and collecting the information of vulnerable people (elderly, infirm, babies, pregnant women) during pre-monsoon time. Using qualitative interviews and public planning documents, Patel ( 2014 ) addressed adaptation strategies in the Surat city. The major flood event of 2006 and its after-effects paved the way for increased public consciousness of flood risk and need for adaptation strategies, leading to major investments by the public and private sector players in adaptation strategies and methods. Micro-level approaches for prevention and response protocol at ward level in addition to city level were also implemented specially for flood risk. Over the years, Surat city has acquired successful adaptation and resilience strategies to deal with extreme events. The ever-changing environmental conditions are a challenge to the city and thus warrant further studies regarding adaptation strategies considering future scenarios.
In Mumbai, more than half of the population live in squatter settlements, rendering this low-income group more vulnerable to the perils of the recurring floods. There was always an inertia in response by the authorities towards extreme events in the city (Johnson et al. 2015 ), which was well reflected in the 2005 Mumbai flood. The devastating flood leads to the constitution of a fact finding committee to find out the causes for the disaster and to take measures. In order to reduce future risks, the government also initiated the Mithi river bed restoration plan. The river is choked with plastic wastes and is devoid of a floodplain because of the encroachment and the illegal settlements. Due to this, the slightest unforeseen rainfall can cause flooding in the region (Vengurlekar and Patkar 2016 ). A public participatory workshop method called Yonmenkaigi System Method (YSM) was implemented in the Mumbai region, which imbibe the concerns and dimensions of the problems based on the perspective of the community (Samaddar et al. 2015 ). The method will enable the community to assess their strengths and weaknesses rather than depending on other supports, such as from civic bodies, for tackling and adapting to the flood-related problems. The adaptation strategies in the Mumbai region should identify the physical, economic and social vulnerability of the areas with poor and low-income households, small businesses and informal settlements, and adaptation should be incorporated as a main component into larger developmental goals (Patankar and Patwardhan 2016 ). Surjan and Shaw ( 2009 ) analysed the role of participatory mechanism called ALM (Advanced Locality Management) in flood risk reduction. ALM has enabled people to care the environment beyond their own household and work for the entire locality. The local government also encouraged this community effort since it reduces flood risks as well as the burden on overstressed municipal activities, which further helps in reducing water-borne diseases and epidemics in the region. These types of people or community-centred activities with the support of government can play a major role in the reduction in disaster hazard risks. Based on the data collected from informal settlements in Mumbai, Chatterjee ( 2010 ) showed different adaptation strategies taken by the population occupying these settlements. These include structural adjustments, support networks after the flood event and networks of loss redistribution. Considering the informal settlements, Subramanyam and MacAlister ( 2016 ) elaborated the prevailing gap in the perception of solutions to flooding risks between the community and local government. Micro-Small and Medium Enterprises (MSMEs), which are some of the most affected establishments during flood events, in the flood-affected areas implemented their own flood protection measures which include various structural and non-structural measures (Schaer and Pantakar 2018 ).
Kolkata’s seasonal flooding has prompted the local population to adapt a number of management strategies. However, challenges are still continuing due to the adaptation deficit in (1) sewer network, (2) drainage infrastructure and (3) financial resources. Some recommended adaptive measures are de-siltation of the existing main sewer, construction of new main sewers, extension of the drainage facility, improvement in the storm water drainage system and renovation of the outfall canal system (Dasgupta et al. 2013 ). A substantial transition in risk governance is observed in the region in recent times, and the flood risks are managed more efficiently and in a responsive mode (Parasuram et al. 2016 ). For example, pumps are deployed immediately after heavy rainfall events to drain the flood water.
In Chennai, urbanization coupled with climate variability turned out as a prominent reason for flood-induced risks, which was well reflected in the recent 2015 flood events. An integrated approach is essential to avert the vagaries of extreme flood events. This approach should combine watershed and land-use management with planning of development, engineering precautions, flood preparedness and emergency management in affected areas (Lavanya 2012 ). Local adaptation strategies are essential to bring about flood resilience in the region (Tajuddin 2018 ), by constructing socio-ecological resilience at the neighbourhood level. Geotagging and text mining techniques were used to understand the resilience of infrastructure using 2015 flood data (Chong et al. 2018 ), and social media is found to be very useful in evaluating the infrastructure resiliency (Yadav and Rahman 2016 ; Gunessee et al. 2017 ) and flood adaptation (Nair et al. 2017 ). Locality-specific, culturally relevant green social work which was implemented in the region proved to be a better adaptation strategy to tackle the flood risks (Samuel et al. 2018 ).
Concluding remarks
This study briefly reviewed the flood risk and adaptation assessment studies in major Indian coastal cities. Four coastal cities (Surat, Mumbai, Chennai and Kolkata) were selected based on the flood risks, anthropogenic activities and vulnerability from flood hazards. This review study also identified some knowledge gaps in flood risk assessment and allied studies in Indian coastal cities. Among the four cities, Mumbai followed by Surat is most vulnerable in terms of flood risks associated with anthropogenic activities. Another factor which exacerbates flood risk in all these cities is the lack of proper drainage and sewerage system. Community-based adaptation strategies in grassroots level supported by local governments show some successes in adaptation for tackling flood risk scenarios in urban areas. Economic minorities are mostly affected by flood risk and associated problems in these cities. Future planning and management of flood risks in these cities should also include economic minorities for the sustainability of city environments. Early warning systems combined with information technology should be effectively implemented in flood risk management and adaptation strategies to make the strategies more effective and practical. Though this review has a drawback that only four cities were considered, the study can be representative of other Indian coastal cities which have similar trends in environmental and demographic settings.
Agnihotri PG, Patel JN (2008) Study of flood at Surat City and its remedial measures the 3rd IASME. In: WSEAS international conference on water resources, hydraulics and hydrology, vol 3, pp 23–25
Agnihotri PG, Patel JN (2011) Improving carrying capacity of River Tapi (Surat, India) by channel modification. Int J Adv Eng Technol 2:231–238
Google Scholar
Ahmed CF, Kranthi N (2018) Flood vulnerability assessment using geospatial techniques: Chennai, India. Indian J Sci Technol 11(6):1–13
Arabindoo P (2016) Unprecedented natures? An anatomy of the Chennai floods. City 20(6):800–821
Bahinipati CS, Rajasekar U, Acharya A, Patel M (2015) Flood-induced economic loss and damage to the textile industry in Surat City, India. Asian Cities climate resilience working paper series 26
Bai X, Dawson RJ, Ürge-Vorsatz D et al (2018) Six research priorities for cities and climate change. Nature 555:23–25. https://doi.org/10.1038/d41586-018-02409-z
Article Google Scholar
Balica SF, Wright NG, van der Meulen F (2012) A flood vulnerability index for coastal cities and its use in assessing climate change impacts. Nat Hazards 64:73–105
Bhat GK, Karanth A, Dashora L, Rajasekar U (2013) Addressing flooding in the city of Surat beyond its boundaries. Environ Urban 25:1–13
Bose S (2008) Adaptive and integrated management of wastewater and storm water drainage in Kolkata—case study of a Mega City. In: Pahl Wostl C, Kabat P, Moltgen J (eds) Adaptive and integrated water management: coping with complexity and uncertainty. Springer, Heidelberg, New York, Berlin, pp 341–355
Boyaj A, Ashok K, Ghosh S, Devanand A, Dandu G (2018) The Chennai extreme rainfall event in 2015: the Bay of Bengal connection. Clim Dynam 50(7–8):2867–2879
Chakraborty T (2010) Flood inundation study of Kolkata Metro City with remote analysis of vulnerability by non-linearization algorithm (RAVANA). Doctoral dissertation, Jadavpur University
Chatterjee M (2010) Slum dwellers response to flooding events in the megacities of India. Mitig Adapt Strateg Glob Change 15:337–353
Chong WK, Naganathan H, Liu H, Ariaratnam S, Kim J (2018) Understanding infrastructure resiliency in Chennai, India using Twitter’s Geotags and texts: a preliminary study. Engineering 4(2):218–223
Courty L (2018) Integrated modelling of overland flows and drainage networks in an urban environment. Thesis
Creel L (2003) Ripple effects: population and coastal regions. Population Reference Bureau, Washington, DC
Cross JA (2001) Megacities and small towns: different perspectives on hazard vulnerability. Gob Environ Change 3:63–80
Da Cunha, Jose G (1902) The origin of Bombay (Vol. 20). Society’s library
Dasgupta S, Gosain AK, Rao S, Roy S, Sarraf M (2013) A megacity in a changing climate: the case of Kolkata. Clim Change 116:747–766
de Oliveira JAP, Doll CN (2016) Governance and networks for health co-benefits of climate change mitigation: lessons from two Indian cities. Environ Int 97:146–154
Drdácký MF (2010) Flood damage to historic buildings and structures. J Perform Constr Fac 24(5):439–445
Drescher A, Glaser R, Pfeiffer C, Vencatesan J, Schliermann-Kraus E, Glaser S, Dostal P (2007) Risk assessment of extreme precipitation in the coastal areas of Chennai as an element of catastrophe prevention. In: Forum DKKV/CEDIM: disaster reduction in climate change
Füssel HM, Klein RJ (2006) Climate change vulnerability assessments: an evolution of conceptual thinking. Clim Change 75:301–329
Ghosh A (2015) Kolkata and climate change. Clim Change Policy Pap IV WWF 4:1–20
Glaser S, Glaser R, Drescher A, Pfeiffer C, Schliermann-Kraus E, Lechner M, Vencatesan J (2008) Geo-communication for risk assessment and catastrophe prevention of flood events in the coastal areas of Chennai. In: Sànchez-Marrè M, Béjar J, Comas J, Rizzoli A (eds) iEMSs 2008: International Congress on Environmental Modelling and Software Integrating Sciences and Information Technology for Environmental Assessment and Decision Making 4th Biennial Meeting of iEMSs. International Environmental Modelling and Software Society
Gokilashree R (2015) A feasibility analysis on new drainage channel to control flooding in Southwest Chennai using hydraulic model. J Water Resour Eng Manag 2(1):1–7
Gunessee S, Subramanian N, Roscoe S, Ramanathan J (2017) The social preferences of local citizens and spontaneous volunteerism during disaster relief operations. Int J Prod Res 16:1–6
Gupta V (1987) Tourism in India. Gyan Publishing House, New Delhi
Gupta K (2007) Urban flood resilience planning and management and lessons for the future: a case study of Mumbai, India. Urban Water J 4(3):183–194
Gupta AK (2009) Urban floods: case study of Mumbai. Disaster Dev 3(2):99
Gupta AK, Nair SS (2010) Flood risk and context of land-uses: Chennai city case. J Geogr Reg Plan 3:365–372
Gupta AK, Nair SS (2011) Urban floods in Bangalore and Chennai: risk management challenges and lessons for sustainable urban ecology. Curr Sci 10:1638–1645
Gupta K, Nikam V (2013) A methodology for rapid inundation mapping for a megacity with sparse data: case of Mumbai, India. IAHS-AISH publication 385-391
Hallegatte S et al (2010) Flood risks, climate change impacts and adaptation benefits in Mumbai: an initial assessment of socio-economic consequences of present and climate change induced flood risks and of possible adaptation options. OECD environment working papers, no. 27. OECD Publishing
Hartter J, Hamilton LC, Boag AE, Stevens FR, Ducey MJ, Christoffersen ND, Oester PT, Palace MW (2018) Does it matter if people think climate change is human caused? Clim Serv 10:53–62
Hunt A, Watkiss P (2011) Climate change impacts and adaptation in cities: a review of the literature. Clim Change 104:13–49
ICOMOS (2015) Cultural heritage, the UN sustainable development goals, and the new urban agenda. In: ICOMOS concept note for the United Nations post-2015 agenda and the third United Nations conference on housing and sustainable urban development (HABITAT III)
IYB (2007) India year book. Government of India, New Delhi
Jaiswal N, Kishtawal CM, Bhomia S (2018) Similarity-based multi-model ensemble approach for 1–15-day advance prediction of monsoon rainfall over India. Theor Appl Climatol 132:639–645. https://doi.org/10.1007/s00704-017-2109-6
Jameson S, Baud IS (2016) Varieties of knowledge for assembling an urban flood management governance configuration in Chennai, India. Habitat Int 54:112–123
Jariwala RC, Samtani BK (2012) The estimation of flood and its control by section modification in Mithi and Kankara tributaries at Surat. Int J Eng Res Appl 2:862–867
Jigyasu R (2016) Reducing disaster risks to urban cultural heritage: global challenges and opportunities. J Herit Manag 1(1):59–67
Joerin J, Shaw R, Takeuchi Y, Krishnamurthy R (2012) Assessing community resilience to climate-related disasters in Chennai, India. Int J Disaster Risk Reduct 1:44–54
Johnson C, Toly N, Schroeder H (eds) (2015) The urban climate challenge: rethinking the role of cities in the global climate regime. Routledge, London
Jongman B (2018) Effective adaptation to rising flood risk. Nat Commun 9(1):1986
Joshi PM, Sherasia NK, Patel DP (2012) Urban flood mapping by geospatial technique a case study of Surat City. IOSR J. Eng 2:43–51
Kale VS, Ely LL, Enzel Y, Baker VR (1996) Palaeo and historical flood hydrology, Indian Peninsula. Geol Soc Lond Spec Publ 115:155–163. https://doi.org/10.1144/GSL.SP.1996.115.01.12
Karambas TV (2015) Modelling of climate change impacts on coastal flooding/erosion, ports and coastal defence structures. Desalin Water Treat 54(8):2130–2137
Kosambi M, Brush JE (1988) Three colonial port cities in India. Geograph Rev 78(1):32–47
Krishnamurthy L, Vecchi GA, Yang X, van der Wiel K, Balaji V, Kapnick SB, Underwood S (2018) Causes and probability of occurrence of extreme precipitation events like Chennai 2015. J Clim 31(10):3831–3848
Kulkarni AT, Mohanty J, Eldho TI, Rao EP, Mohan BK (2014) A web GIS based integrated flood assessment modeling tool for coastal urban watersheds. Comput Geosci 64:7–14
Kumar R, Jawale P, Tandon S (2008) Economic impact of climate change on Mumbai, India. Reg Health Forum 12:38–42
Lavanya AK (2012) Urban flood management—a case study of Chennai City. Archit Res 2:115–121
Lindley SJ, Handley JF, Theuray N, Peet E, McEvoy D (2006) Adaptation strategies for climate change in the urban environment: assessing climate change related risk in UK urban areas. J Risk Res 9:543–568
Mahmood S, Davie J, Jermey P et al (2018) Indian monsoon data assimilation and analysis regional reanalysis: configuration and performance. Atmos Sci Lett 19:3. https://doi.org/10.1002/asl.808
Mariappan VN, Devi RS (2012) Chennai coast vulnerability assessment using optical satellite data and GIS techniques. Int J Remote Sens 1:175–182
Marzeion B, Levermann A (2008) Loss of cultural world heritage and currently inhabited places to sea-level rise. Environ Res Lett 9(3):034001
Mathew S, Trück S, Henderson-Sellers A (2012) Kochi, India case study of climate adaptation to floods: ranking local government investment options. Glob Environ Change 22(1):308–319
MCGM (2014) Greater Mumbai city development plan 2005–2025. Municipal Corporation of Greater Mumbai, Mumbai
Mendelsohn R, Emanuel K, Chonabayashi S, Bakkensen L (2012) The impact of climate change on global tropical cyclone damage. Nat Clim Change 2(3):205
Mishra AK (2016) Monitoring Tamil Nadu flood of 2015 using satellite remote sensing. Nat Hazards 82(2):1431–1434
Muneerudeen A (2017) Urban and landscape design strategies for flood resilience in Chennai city. Master’s thesis
Murphey R (1964) The city in the swamp: aspects of the site and early growth of Calcutta. Geogr J 130:241–256
Nair MR, Ramya GR, Sivakumar PB (2017) Usage and analysis of Twitter during 2015 Chennai flood towards disaster management. Procedia Comput Sci 115:350–358
NDMA (2017) National disaster management guidelines for cultural heritage sites and precincts, September 2017. New Delhi A publication of the National Disaster Management Authority, Government of India
Nicholls RJ, Small C (2002) Improved estimates of coastal population and exposure to hazards released. Eos 83:301–305
Nicholls RJ et al (2008) Ranking port cities with high exposure and vulnerability to climate extremes: exposure estimates. OECD environment working papers, no. 1. OECD Publishing
Pacione M (2006) City profile Mumbai. Cities 23:229–238
Parasuram P, Narayanan P, Pelling M, Solecki W, Ramachandran P, Ramachandran R (2016) Climate change adaptation pathways in Kolkata. J Extreme Events 3(03):1–22
Parikh K, Parikh J, Kumar M (2017) Vulnerability of Surat, Gujarat to flooding from Tapi River: a climate change impact assessment. Vayu Mandal 43(2):120–129
Patankar A, Patwardhan A (2016) Estimating the uninsured losses due to extreme weather events and implications for informal sector vulnerability: a case study of Mumbai, India. Nat Hazards 80(1):285–310
Patankar A, Patwardhan A, Andharia J, Lakhani V (2010) Mumbai city report. In: International workshop on climate change vulnerability assessment and urban development planning for Asian coastal cities
Patel T (2014) Funding for adaptation to climate change: the case of Surat. Doctoral dissertation, Massachusetts Institute of Technology
Patel DP, Dholakia MB (2010) Identifying probable submergence area of Surat City using digital elevation model and geographical information system. World Appl Sci J 9(4):461–466
Patel DP, Ramirez JA, Srivastava PK et al (2017) Assessment of flood inundation mapping of Surat city by coupled 1D/2D hydrodynamic modeling: a case application of the new HEC-RAS 5. Nat Hazards 89:93–130. https://doi.org/10.1007/s11069-017-2956-6
Pavri F (2010) Urban expansion and sea-level rise related flood vulnerability for Mumbai (Bombay), India using remotely sensed data. In: Showalter P, Lu Y (eds) Geospatial techniques in urban hazard and disaster analysis. Springer, Dordrecht, pp 31–49
Pramanik MK (2017) Impacts of predicted sea level rise on land use/land cover categories of the adjacent coastal areas of Mumbai megacity, India. Environ Dev Sustain 19(4):1343–1366
Rahmstorf S (2017) Rising hazard of storm-surge flooding. Proc Natl Acad Sci 114(45):11806–11808
Ramasamy SM, Vijay A, Dhinesh S (2018) Geo-anthropogenic aberrations and Chennai floods: 2015, India. Nat Hazards 92(1):443–477
Ramesh C, Vivekanandan N, Surwade KB, Bapat AD, Govindan S, Mathew FT (2008) Extreme value analysis of rainfall in Mumbai region to aid estimation of severe flood. ISH J Hydraul Eng 14(2):102–117
Ramirez J, Rajasekar U (2015) Modelling flood impact in Surat, India. In: TIFAC-IDRiM conference, New Delhi, India
Ramirez J, Rajasekar U, Coulthard T, Keiler M (2016) Probabilistic mapping of urban flood risk: application to extreme events in Surat, India. In: EGU general assembly conference abstracts, vol 18, p 2093
Ranger N, Hallegatte S, Bhattacharya S, Bachu M, Priya S, Dhore K, Corfee-Morlot J (2011) An assessment of the potential impact of climate change on flood risk in Mumbai. Clim Change 104:139–167
Rawlinson HG (1920) British beginnings in Western India, 1579–1657: an account of the early days of the British factory of Surat. Clarendon Press, Oxford
Revi A (2008) Climate change risk: an adaptation and mitigation agenda for Indian cities. Environ Urban 20:207–229
Rodriguez JA, Fernandez FJ, Arboleya P (2018) Study of the architecture of a smart city. Proceedings 2(23):1485
Rossnerova A, Pokorna M, Svecova V, Sram RJ, Topinka J, Zölzer F, Rossner P (2017) Adaptation of the human population to the environment: current knowledge, clues from Czech cytogenetic and “omics” biomonitoring studies and possible mechanisms. Mutat Res Rev Mutat 773:188–203
Rumbach A (2014) Do new towns increase disaster risk? Evidence from Kolkata, India. Habitat Int 43:117–124
Rumbach A, Shirgaokar M (2017) Predictors of household exposure to monsoon rain hazards in informal settlements. Nat Hazards 85(2):709–728
Samaddar S, Chatterjee R, Misra BA, Tatano H (2011) Participatory risk mapping for identifying spatial risks in flood prone slum areas, Mumbai. Annu Disaster Prev Res Inst Kyoto Univ 54B
Samaddar S, Choi J, Misra BA, Tatano H (2015) Insights on social learning and collaborative action plan development for disaster risk reduction: practicing Yonmenkaigi system method (YSM) in flood-prone Mumbai. Nat Hazards 75(2):1531–1554
Samuel M, Annadurai P, Sankarakrishnan S (2018) The 2015 Chennai floods: green social work, an emerging model for practice in India. The Routledge handbook of green social work, pp 281–292
Schaer C, Pantakar A (2018) Promoting private sector engagement in climate change adaptation and flood resilience—a case study of innovative approaches applied by MSMEs in Mumbai, India. In: Alves F, Leal Filho W, Azeiteiro U (eds) Theory and practice of climate adaptation. Springer, pp 175–191
Schaer C, Bee S, Kuruppu N (2018) Developing the business case for adaptation in agriculture: case studies from the adaptation mitigation readiness project. Private-sector action in adaptation: perspectives on the role of micro, small and medium size enterprises, p 51
Seenirajan M, Natarajan M, Thangaraj R, Bagyaraj M (2017) Study and analysis of Chennai flood 2015 using GIS and multicriteria technique. J Geogr Inf Syst 9(02):126
Sekovski I, Newton A, Dennison WC (2012) Megacities in the coastal zone: using a driver-pressure-state-impact-response framework to address complex environmental problems. Estuar Coast Shelf Sci 96:48–59
Selvaraj K, Pandiyan J, Yoganandan V, Agoramoorthy G (2016) India contemplates climate change concerns after floods ravaged the coastal city of Chennai. Ocean Coast Manage 129:10–14
Sen D (2013) Real-time rainfall monitoring and flood inundation forecasting for the city of Kolkata. ISH J Hydraul Eng 19(2):137–144
Shahapure SS, Eldho TI, Rao EP (2011) Flood simulation in an urban catchment of Navi Mumbai City with detention pond and tidal effects using FEM, GIS, and remote sensing. J Waterw Port Coast Ocean Eng 137:286–299
Shahi NK, Rai S, Mishra N (2018) Recent predictors of Indian summer monsoon based on Indian and Pacific Ocean SST. Meteorol Atmos Phys. https://doi.org/10.1007/s00703-018-0585-6
Sharma VK, Mishra N, Shukla AK, Yadav A, Rao GS, Bhanumurthy V (2017) Satellite data planning for flood mapping activities based on high rainfall events generated using TRMM, GEFS and disaster news. Ann GIS 23(2):131–140
Singh PD, Kambekar AR (2017) Assessing impact of sea level rise along the coastline of Mumbai City using geographic information system. In: Seta F, Biswas A, Khare A, Sen J (eds) Understanding built environment. Springer, pp 87–96
Singh AK, Sharma AK (2009) GIS and a remote sensing-based approach for urban flood-plain mapping for the Tapi catchment, vol 331. IAHS Publication, Wallingford, p 389
Small C, Nicholls RJ (2003) A global analysis of human settlement in coastal zones. J Coast Res 19:584–599
SMC (2011) Surat City resilience strategy. Surat Municipal Corporation, Surat
Solecki W, Rosenzweig C, Dhakal S et al (2018) City transformations in a 1.5 °C warmer world. Nat Clim Change 8:177–181
Stephen A (2016) Natural disasters: Are they really natural? In: Proceedings of ICSSR sponsored national seminar on infrastructural development and environment: issues and challenges
Subramanian R (2012) Implementation of neural networks in flood forecasting. Int J Sci Res Publ 2(10):1–3
Subramanyam N, MacAlister C (2016) Governance of flooding risks in informal settlements in satellite cities in the Mumbai region. South Asian Water Stud 6(1):12–27
Suriya S, Mudgal BV (2012) Impact of urbanization on flooding: the Thirusoolam sub watershed—a case study. J Hydrol 412:210–219
Suriya S, Mudgal BV, Nelliyat P (2012) Flood damage assessment of an urban area in Chennai, India, part I: methodology. Nat Hazards 62:149–167
Surjan A, Shaw R (2009) Enhancing disaster resilience through local environment management: case of Mumbai, India. Disaster Prev Manage 18:418–433
Taboroff J (2003) Natural disasters and urban cultural heritage: a reassessment. In: Kreimer A, Arnold M, Carlin A (eds) Building safer cities: the future of disaster risk. The World Bank, Disaster Risk Management, Series no. 3, 2003, pp. 233–240
Tajuddin N (2018) Leveraging socio-cultural networks: local adaptation strategies to bring about flood resilience in Chennai metropolitan area, India. Thesis TU Delft Architecture and the Built Environment
UN (2008) Urban agglomerations 2007. United Nations Department of Economic and Social Affairs, Population Division, New York
UNDESA (2016) The world’s cities in 2016. United Nations, Department of Economic and Social Affairs, Population Division, New York, p 392
UNESCO (2009) World water assessment programme (United Nations). Water in a changing world. UNESCO, Paris
Ürge-Vorsatz D, Rosenzweig C, Dawson RJ et al (2018) Locking in positive climate responses in cities. Nat Clim Change 8:174–177
Van Oldenborgh GJ, Otto FE, Haustein K, AchutaRao K (2016) The heavy precipitation event of December 2015 in Chennai, India. Bull Am Meteorol Soc 97(12):87–91
Vaz E (2014) Managing urban coastal areas through landscape metrics: an assessment of Mumbai’s mangrove system. Ocean Coast Manage 98:27–37
Vengurlekar M, Patkar S (2016) Mithi river regeneration. International Journal of Research in Advent Technology, Special Issue RDACE, March 2016, 90–96, Special Issue National Conference VishwaCon’16, 19 March 2016
VishnuRadhan R, Vethamony P, Zainudin Z, Kumar KV (2014) Waste assimilative capacity of coastal waters along Mumbai Mega City, West Coast of India using MIKE-21 and WASP simulation models. CLEAN 42:295–305
Vitousek S, Barnard PL, Fletcher CH et al (2017) Doubling of coastal flooding frequency within decades due to sea-level rise. Sci Rep 7:1399
Vojinovic Z, Hammond M, Golub D, Hirunsalee S, Weesakul S, Meesuk V, Medina N, Sanchez A, Kumara S, Abbott M (2016) Holistic approach to flood risk assessment in areas with cultural heritage: a practical application in Ayutthaya, Thailand. Nat Hazards 81(1):589–616
Von Glasow R, Jickells TD, Baklanov A, Carmichael GR, Church TM, Gallardo L, Zhu T (2013) Megacities and large urban agglomerations in the coastal zone: interactions between atmosphere, land, and marine ecosystems. Ambio 42:13–28
Wilby RL, Keenan R (2012) Adapting to flood risk under climate change. Prog Phys Geogr 36(3):348–378
World Bank (2011) Vulnerability of Kolkata metropolitan area to increased precipitation in a changing climate environment, climate change and water resources department South Asia region. World Bank, Washington, DC
Yadav M, Rahman Z (2016) The social role of social media: the case of Chennai rains-2015. Soc Netw Anal Min 6(1):101
Zope PE, Eldho TI, Jothiprakash V (2015) Impacts of urbanization on flooding of a coastal urban catchment: a case study of Mumbai City, India. Nat Hazards 75(1):887–908
Zope PE, Eldho TI, Jothiprakash V (2016) Impacts of land use–land cover change and urbanization on flooding: a case study of Oshiwara River Basin in Mumbai, India. CATENA 145:142–154
Download references
Acknowledgements
RD acknowledges the Ministry of Human Resource Development (MHRD) of Govt. of India for providing funding under Teaching Assistantship category. RVR acknowledges SERB-National Post-Doctoral fellowship (PDF/2017/002093) from Science and Engineering Research Board, Department of Science and Technology, Government of India. The authors thank Dr. Rohit Jigyasu, President, ICOMOS India, and members of National Scientific Committee, Risk Preparedness, ICOMOS India, for inputs.
Author information
Ravinder Dhiman and Renjith VishnuRadhan have contributed equally to this work.
Authors and Affiliations
Centre for Urban Science and Engineering, Indian Institute of Technology Bombay, Mumbai, 400076, India
Ravinder Dhiman
Department of Civil Engineering, Indian Institute of Technology Bombay, Powai, Mumbai, Maharashtra, 400076, India
Renjith VishnuRadhan & T. I. Eldho
Centre of Studies in Resources Engineering, Indian Institute of Technology Bombay, Mumbai, 400076, India
Arun Inamdar
You can also search for this author in PubMed Google Scholar
Corresponding author
Correspondence to Renjith VishnuRadhan .
Additional information
Publisher’s note.
Springer Nature remains neutral with regard to jurisdictional claims in published maps and institutional affiliations.
Rights and permissions
Open Access This article is distributed under the terms of the Creative Commons Attribution 4.0 International License ( http://creativecommons.org/licenses/by/4.0/ ), which permits unrestricted use, distribution, and reproduction in any medium, provided you give appropriate credit to the original author(s) and the source, provide a link to the Creative Commons license, and indicate if changes were made.
Reprints and permissions
About this article
Dhiman, R., VishnuRadhan, R., Eldho, T.I. et al. Flood risk and adaptation in Indian coastal cities: recent scenarios. Appl Water Sci 9 , 5 (2019). https://doi.org/10.1007/s13201-018-0881-9
Download citation
Received : 03 July 2016
Accepted : 22 November 2018
Published : 04 December 2018
DOI : https://doi.org/10.1007/s13201-018-0881-9
Share this article
Anyone you share the following link with will be able to read this content:
Sorry, a shareable link is not currently available for this article.
Provided by the Springer Nature SharedIt content-sharing initiative
- Flood management
- Urban planning
- Coastal megacities
- Find a journal
- Publish with us
- Track your research
- Reference Manager
- Simple TEXT file
People also looked at
Review article, floods and flood management and its socio-economic impact on pakistan: a review of the empirical literature.

- 1 Department of Govt and Public Policy, Faculty of Contemporary Studies, National Defence University, Islamabad, Pakistan
- 2 Department of Earth and Environmental Sciences, Bahria University, Islamabad, Pakistan
- 3 School of Economics, Sapienza University of Rome, Rome, Italy
- 4 Department of Environmental Sciences, Faculty of Life Sciences and Informatics, Balochistan University of Information Technology, Engineering and Management Sciences, Quetta, Pakistan
- 5 School of Public Administration, Xiangtan University, Hunan, China
- 6 School of Public Administration, China University of Geosciences, Wuhan, China
- 7 The Faculty of Biosciences, Fisheries and Economics, UiT The Arctic University of Norway, Tromsø, Norway
- 8 Department of Earth Sciences, Faculty of Sciences, Ibn Zohr University, Agadir, Morocco
- 9 MARE-Marine and Environmental Sciences Centre—Sedimentary Geology Group, Department of Earth Sciences, Faculty of Sciences and Technology, University of Coimbra, Coimbra, Portugal
Flood is one of the most damaging natural disasters as the recent floods have shown their serious impact on Pakistan. Flood control and regulation policies are essential to reduce the risks of economic downturn, a threat to human existence, and to sustain the ecology. The severity of flood catastrophe activities represents a constant and severe issue in the world. Floods are rising year by year in severity and duration, causing negative impacts on the social and economic conditions of the nation concerned. While the frequency of floods cannot be avoided, their adverse impacts can be considerably reduced by adopting careful planning and efficient training. This paper reviews the socioeconomic impact of floods, and the existing condition of flood control policies outlines the flood protection problems and discusses opportunities for successful and efficient flood control in Pakistan. The paper also intends to propose several suggestions for efficient and sustainable flood control in Pakistan.
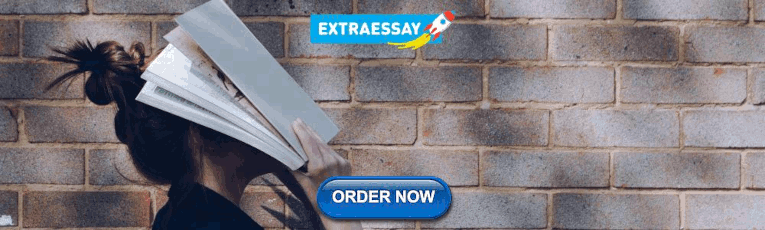
1 Introduction
Floods are one of the major sources of anthropological and ecological destruction. It affects the socio-economic conditions, worsens public health, generates unemployment, damages the ecosystem, etc. ( Allaire, 2018 ; Parida, 2019 ). Currently, public and private institutions are struggling to formulate and evaluate risk management and adjustment strategies, involving systems for flood prevention and advance alerts with urbanization patterns and land use planning under consideration in the wake of urban flooding. One major reason is the land adjudication and administration system of Pakistan is colonial in nature and lacks judicial augmentation, providing a chance for flawed urbanization ( Shafi et al., 2022 ). However, policymakers face substantial hiccups in the mitigation of natural disasters’ aftermath globally. In this wake, countries with stable economic structures and administration have reported fewer mortalities and lower socioeconomic damages as compared to developing countries ( Anbarci et al., 2005 ; Kahn, 2005 ).
Certain approaches are utilized to manage floods and mitigate their aftermath. Here, Effective Risk Avoidance involves a detailed understanding of the effects of floods on the public, the economy, and the efficiency of disaster management strategies. The realistic approaches focus on the potential risks and benefits analysis. Currently, prevention approaches are often decided based on active expert analysis of floods. However, cost-benefit analysis is seldom used in mitigation planning, possibly because of insufficient empirical evidence on a broad range of types of losses. Government and non-governmental sectors also transfer their resources from production to restoration and rehabilitation practices which slow down the growth of Gross Domestic Product (GDP) and overall Human Development Index (HDI) ( Sadia et al., 2013 ; Isik et al., 2021 ).
The South-Asian Subcontinent, with The Great Himalaya Glaciers, remains at primary risk. Though currently, the rainy season remains the major reason for the flooding in the region the presence of glaciers and the rise in average temperature remain a permanent threat to the region. Floods are the most common natural disaster in the region with 40% occurrence rate ( Chaudhry, 2017 ). Furthermore, urban flooding reasoned by solid waste management and decreases in urban vegetation has affected 9.6 million people in Bangladesh, India, and Nepal, with 6.8 million from India only. South Asia floods: 9.6 million people swamped as humanitarian crisis deepens ( IFRC, 2020 ).
It has been observed that the average global temperature has been rising at a higher rate since 1980. Due to this glaciers are melting rapidly, generating glacial lakes and associated hazards. Glacial Lake Outburst Floods (GLOFs) are extremely destructive because of large volumes of water flowing in narrow river channels. The greatest number of GLOFs, out of all the natural disasters that have occurred worldwide, have been reported in Central Asia ( Carrivick and Tweed, 2016 ; Nie et al., 2017 ; Mohanty and Maiti, 2021 ). Global warming as the sole reason GLOFS, the Hindukush-Karakoram-Himalaya regions of Pakistan, which contribute more than 50% of the entire flow of the Indus River System (a major water system), has observed a higher melting rate of glaciers. This condition has led to an increase in the frequency of glacial-related hazards in this Himalayan region. GLOFs which are either caused by the sudden failure of the “dams,” or in the absence of the dams release huge volumes of water and debris wreak havoc downstream. GLOFs have the potential to massively harm people living in the Himalayan region, especially Indus River Basin ( Ashraf et al., 2012 ; Ashraf et al., 2021 ; Ahmad et al., 2022 ).
Achieving sustainability in the country in terms of economic, social, political, and environmental issues is discussed more thoroughly nowadays because the phenomena of globalization as well as global warming are based to meet today’s requirements without compromising the ability of future generations ( Işık et al., 2021 ). The rising CO2 emission worldwide will be rising government spending as the result increases real GDP per capita. So, state policymakers are trying to limit or minimize CO2 emissions ( Işık et al., 2022 ). Pakistan is among the most vulnerable countries to floods and water-related disasters as Pakistan has the most glaciers outside the arctic circle. The climate changes and monsoon season have significant impacts on socio-economic degradation, specifically on agricultural production and livestock. The regular occurrence of flood catastrophes affects different regions of Pakistan (See Table 1 ). Floods are expensive environmental disasters, leading to property and agricultural land destruction. Floods are typically short-lived occurrences that can occur with a tiny alert ( Commission, 2007 ). Pakistan has experienced an unprecedented increase in floods in the last 20 years. Fifty-four (54) floods of differing intensity hit Pakistan, placing it 10th on Global Environment Risk Index ( Kreft et al., 2015 ; Rehman et al., 2015 ; Sardar et al., 2016 ). Pakistan is positioned within a hazard-prone region and is bare to a variety of natural catastrophes like floods ( Rafiq and Blaschke, 2012 ). The past of the region’s flooding is relatively lengthy. Many significantly disastrous floods caused considerable harm to economic development. Table 2 provides the history of floods in terms of losses incurred. Each flood is caused by heavy rainfall in the Indus River catchments and its main tributaries ( GoP Annual Flood Report, 2017 ). According to the World Health Organization (WHO) twenty (20) million residents in seventy-eight (78) areas were affected by 2010 floods, taking one thousand eight hundred (1800) life’s, causing damage, or destruction of nearly two million houses with a cumulative cost of $9.7 billion. Public infrastructure i.e., roads, bridges, hospitals, schools etc. , were immensely affected, deteriorating accessibility to health, education and mobility resulting in poverty, deprivation, and psychological and social trauma necessitating rehabilitation and restoration ( Sardar et al., 2016 ).
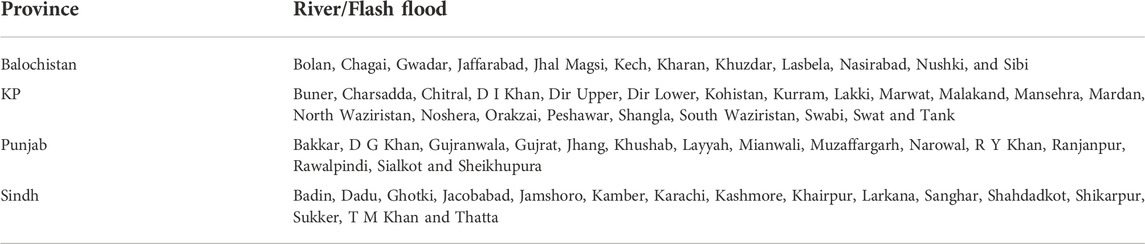
TABLE 1 . Most vulnerable districts of Pakistan for flood and flash flood ( NDMA, 2019 ).
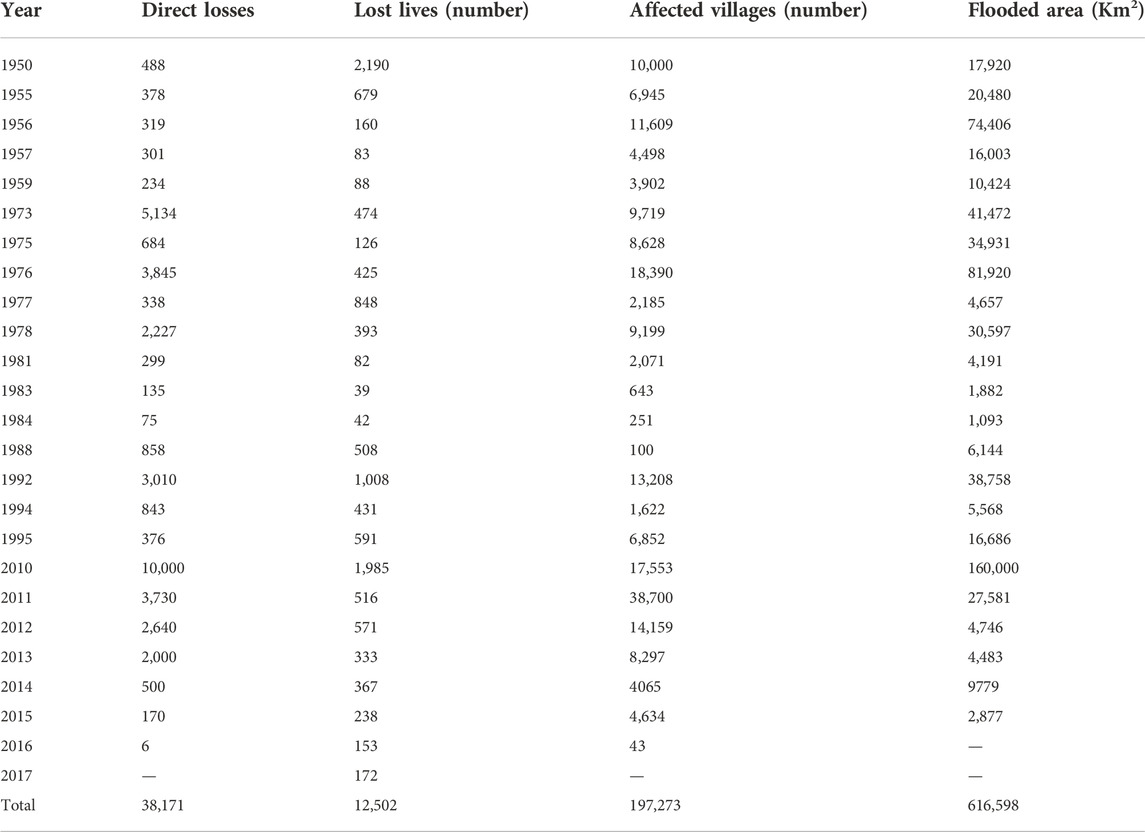
TABLE 2 . Historical flood damages in Pakistan (period 1950–2017) ( GoP Annual Flood Report, 2017 ).
There are typically five forms of flooding frequently occurring in the country, such as flash floods, river floods, tidal floods, marine floods, and pluvial floods. The heavy rainfall in canals’ catchment areas triggers pluvial floods of canals or dams, and water goes out towards the dry land and affects the area ( Yaqub et al., 2015 ). Main floods in Pakistan are linked with the low depression monsoon rainfalls that form in the Bay of Bengal and spread west/northwest through India to Pakistan ( Kronstadt, 2010 ). Apart from Monsoon rains, meltdown of glaciers causes flashfloods in hilly areas rendering massive destruction. However, urban flooding is solely related to monsoon rains and unplanned urbanization restricting drainage passages and encroachments. Furthermore, coastal floods are mostly caused by tropical storms in South-East Sindh and Makran regions ( Yaqub et al., 2015 ). Pakistan faces severe floods from July to September period owing to heavy monsoon rains in all the regions of Pakistan inundating the Indus River Basin. Hydrologically, the region can be categorized into three main divisions: Indus Basin, Kharan Basin, and Makran Coastal Drainage Zone. Such basin’s flooding features differ significantly and require a detailed understanding ( Tariq and van de Giesen, 2012 ). The flood hazard map indicates the most vulnerable districts of Pakistan with major river systems (developed after ( NDMA, 2019 ) as shown in Figure 1 . A topographic map of Pakistan with recent flood events is shown in Figure 2 . The list of the most vulnerable districts of Pakistan concerning Flood and Flash Flood is shown in Table 1 . The geographical distribution of Pakistan’s 2010 floods’ direct and indirect damages is shown in Figure 3 and Figure 4 respectively.
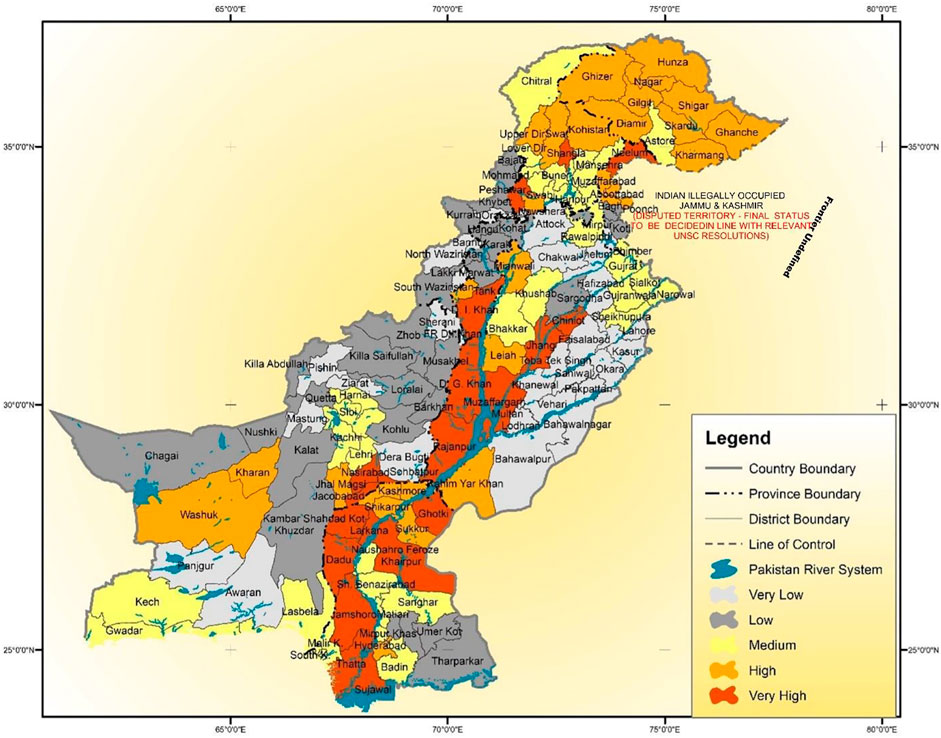
FIGURE 1 . Flood hazard map indicates the most vulnerable districts of Pakistan with a major river system map developed after ( NDMA, 2019 ).
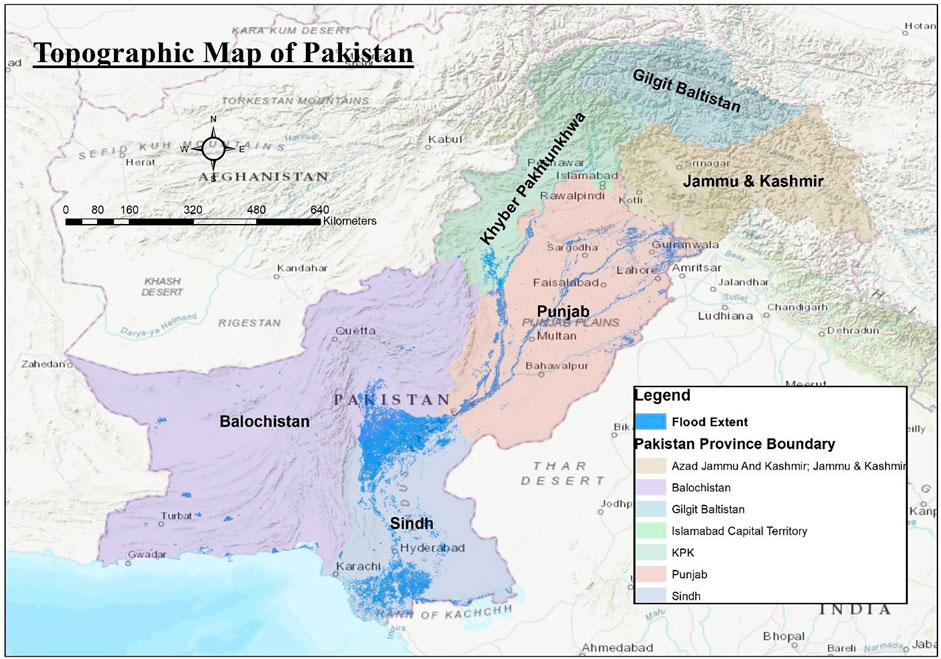
FIGURE 2 . Topographic map of Pakistan with recent flood events (Source: ESRI topographic layer used).
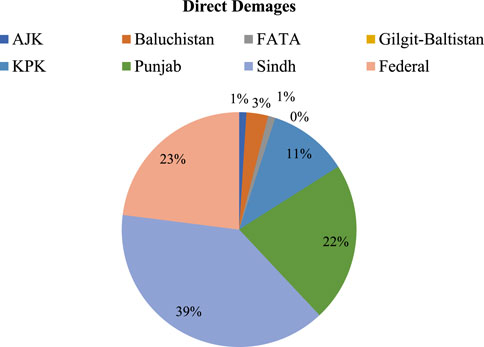
FIGURE 3 . Geographical distribution of Pakistan’s 2010 floods direct damages; Source: Pakistan floods 2010 preliminary damage and needs assessment ( Asian Development Bank, 2010 ).
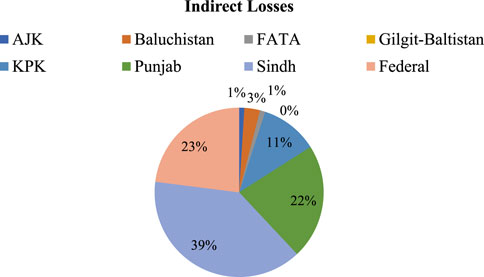
FIGURE 4 . Geographical distribution of Pakistan’s 2010 floods indirect damages; Source: Pakistan floods 2010 preliminary damage and needs assessment ( Asian Development Bank, 2010 ).
Global Warming and climate change are serious issues, concerning Pakistan. Our article represents the impact of global warming and climate change. As evident the cloud bursting phenomenon is quite new for Pakistan, the same is the case with Glacier Bursts. Keeping floods as the focal point of the study we maintain this proposition that climate change is one of the primary reasons for wreaking havoc in Pakistan. With this background, this article examines the recent and emerging developments in catastrophe situations to evaluate the effects of floods on the socio-economic conditions of the higher flood-risk areas. The purpose of this study is to assess the socioeconomic cost of floods and to strengthen flood control policies in Pakistan, which is a resource-limited and vastly populated developing nation, and highly dependent on the agricultural sector. The paper utilizes a literature synthesis approach, extracting data from various resources ranging from published articles to government documents. Moreover, ESRI topographic layer was used to develop a topographic map of Pakistan. The study emphasizes that exploration of flood mitigation criteria is also needed as the floods in the country are becoming more regular and severe.
2 Material and methodology
This study reviews the existing literature on the flood, flood management, and socioeconomic cost in Pakistan focusing on riverine and extreme floods (2010). The theoretical framework of the study is shown in Figure 5 .
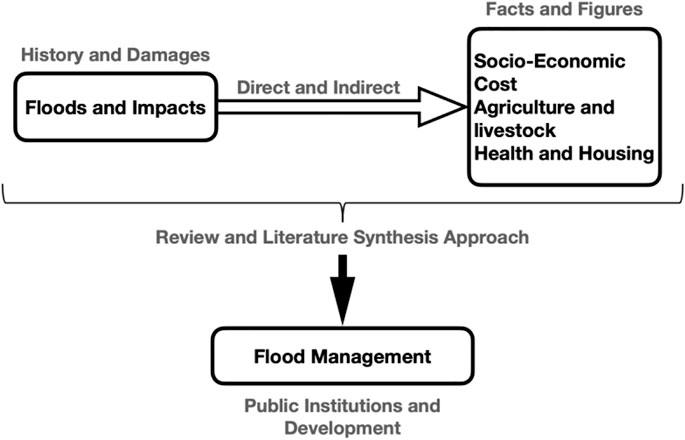
FIGURE 5 . Theoretical framework of the study.
The present study compiled and comprehensively analyzed numerous scientific and analytical reports, scholarly articles relevant to flood management studies, economic surveys of Pakistan, and other relevant reports released by different scholarly, consulting, and consultant institutions to extract and synthesize main studies on floods and flood management in Pakistan. A comprehensive empirical literature review was performed by using Google Scholar and a related search engine. The literature that has the greatest relevance to Pakistan was cited. The study also reported crucial findings and suggestions.
The past research suggests various review methods, empirical analysis, and meta-analysis ( Toya and Skidmore, 2007 ; Jonkman et al., 2008 ; McMullen and Lytle, 2012 ). The studies aim to create an empirical link by collecting data such as the impact of floods on GDP ( Kirigia et al., 2004 ; Rufat et al., 2015 ). But in the case of Pakistan, the data available is insufficient to either adopt or develop any complicated method, however empirical analysis of the facts and figures provided through reports, government documents, and policy papers, and correlate these with studies reviewed. The focus of this study remains to provide insight into the flood problem by reviewing it through data synthesis and textual analysis approach, which will access the impacts of floods. This study will establish an understanding of the importance of the topic, where impact analysis will ensure the need for extended studies conducted in the Netherlands and Africa ( Kirigia et al., 2004 ; Jonkman et al., 2008 ).
3 Floods and their impacts
Our study will steer through past research which includes a broad range of topics concerning measuring the impact of floods i.e., agriculture and livestock, impact on human health, the economic cost of floods, flood forecasting, flood warning mechanisms, and flood management.
As, the recent floods have devastated the country, and have inundated more than 40% of Pakistan, we can only speculate the losses in wholistic terms. It has been recorded that more than 30 million people have been displaced, with most parts of the Sindh province remaining under imminent threat. So currently, the estimates can only be compared in terms of Macro losses, like no. of people migrated, an area inundated, infrastructure destruction, etc. but the exact figures will remain ambiguous. As far as the scale and scope of the current flood are concerned, we can only conclude this fact that it has immensely damaged the country as compared to the flood of 2010.
3.1 Impact on agriculture and livestock
The impact of the flood on the agriculture sector can be divided into the following six categories: 1) Livestock evacuation in an urgent situation; 2) Avoidance of spring field exposure, allowing livestock to be sheltered or moved to certain other flood-free places; 3) Harm to crop and grass productivity in worst-affected areas with massive loss of pasture 4) Driven production loss and affected the performance of cultivation and agricultural land; 5) Destroyed irrigation structures and facilities at the farm; 6) Loss of advantageous soil invertebrates, in particular earthworms, elevated risk of animal disease, including infection of liver fluke ( Morris and Brewin, 2014 ). As agriculture remains the largest sector of Pakistan’s economy, employing 43 percent of the population, its yearly contribution to the GDP period 1969–2011 is shown in Figure 6 .
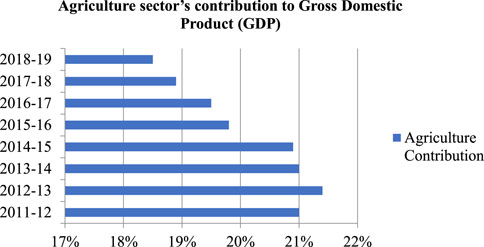
FIGURE 6 . Agriculture sector’s contribution to gross domestic product (GDP) period 2011–2019 (Economic Survey of Pakistan 2018–2019) ( Gop Pakistan Economic Survey, 2019 ).
Climate change typically has minor effects on the world food supply, but the consequences of climate change are widely distributed unevenly. Low-income economies, such as those in South Asia and Africa, experienced most of the casualties. By taking the data from 1989 to 2015 and by using the Feasible General Least Square (FGLS) model ( Ali et al., 2017 ) studied the climate changes impact on Pakistan’s major crop yields. The results showed that except for wheat, the impact of rainfall on the production of any other selected crop is negative ( Iqbal et al., 2018 ). Evaluated the impact of Pakistan’s 2010 flood on Khyber Pakhtunkhwa’s agriculture sector and found that after the flood, the family income of agricultural labor dropped, resultantly, in the usage of chemical fertilizers and other agricultural inputs also declined. The floods have adversely affected agricultural output where there was a considerable fall in wheat, maize, and sugar cane output. In Pakistan, the floods and heavy rains have not only affected agriculture crops, livestock, and forests but also devastated necessary facilities such as tube wells, domestic water storage facilities, animal shelters, private seeds inventories/pesticides, and agricultural equipment ( Iqbal et al., 2018 ). Baluchistan and KPK experienced mostly heavy rains, while Punjab and Sindh experienced mostly slow-rising floods on the canal. 2011, 2012, 2013, and 2014s floods damaged residential and agricultural properties, livestock, and crops. ( Ashraf et al., 2013 ). proposed that floods made food shortages and food insecurity for the citizens as they had to utilize polluted resources, particularly water.
Due to the 2010s flood, the agriculture industry has suffered a total loss of about Rs. 429 billion. For example, the profitability of cotton production decreased to 11.76 million bales as compared to the expected production of 14 million bales. Rates of the inputs of agricultural products such as urea, and chemicals, diesel had increased dramatically ( Bukhari and Rizvi, 2017 ). According to ( Rehman et al., 2015 ), twenty percent of the country’s overall landmass was inundated, affected by the 2010s flood, with cumulative damages of above USD 10 billion. The agriculture sector’s contribution to GDP is shown in Table 3 showing a significant decrease in the fiscal year 2010–11.
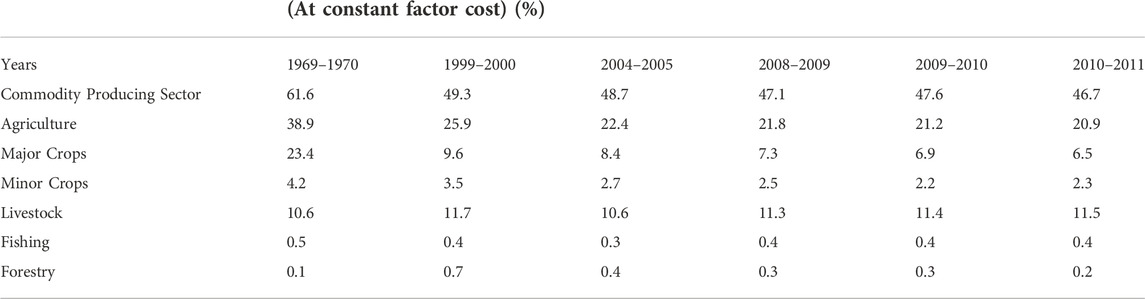
TABLE 3 . Agriculture sector’s contribution to gross domestic product (GDP) period 1969–2011 (Economic survey of Pakistan, 2010–2011) ( GoP Economic Survey, 2011 ).
The flood caused the loss of millions of livestock including around 200,000 dead in the 2010 flood in Pakistan. The final number of losses was higher. The Food and Agriculture Organization (FAO) announced that after the disaster, millions of surviving animals were confronting a food shortage situation that was alarming. The call for 5.7 million dollars has been made by UNO for emergency assistance for livestock. However, funds of 1.4 million dollars have been mobilized by FAO to secure feedstuff and the healthcare of livestock. Currently, the full scale and scope of the catastrophe are not clear, which will require more resource allocations once the situation becomes clearer ( Deen, 2015 ).
3.2 Impact on human health
Floods pose an enormous challenge to the healthcare system and its efficacy. For example, it can damage access to drinkable water by infiltrating the aquifers, thus increasing the transmission of waterborne diseases. The health concerns are classified as direct and indirect. Where direct effects arise from deep water and flooding penetration, including death, debris injury, environmental pollution, and hypothermia. Indirect effects include threats related to the water disruption to the natural and physical environment, including communicable diseases, obesity, famine-related diseases, and displaced population-related diseases. The number of people affected by the different diseases by the 2010’s flood is shown in Figure 7 ( Ahern et al., 2005 ; Du et al., 2010 ).
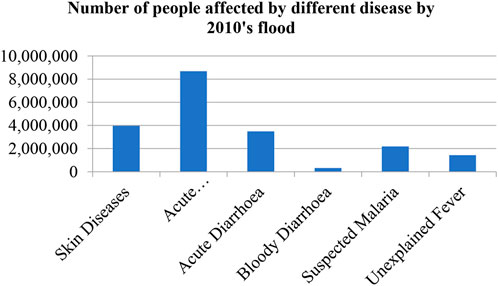
FIGURE 7 . Number of people affected by the different diseases by the 2010’s flood ( GoP, 2011 ).
The floods’ health effects could also be categorized as instantaneous medium and long-term. Flooding can also develop a high number of breeding grounds for insect and infection-borne diseases such as malaria. There have been various reports of increased risk throughout Asia, Africa, and Latin America in historically tropical countries. Public medical professionals and relief workers also warn after natural disasters that the dead bodies of affected people can trigger disease outbreaks such as cholera. The anxiety induced by such statements enables societies, local governments, and institutions to dispose of affected people quickly without identification. This leads to psychological stress for family members alive and causes legal issues including land, insurance claims, and inherited wealth ( Kondo et al., 2002 ; Morgan et al., 2005 ).
Reacher et al. (2004) evaluated the impact of floods on public health by taking the data of massive floods for the period of 12 October 2000 in the region of Lewes in the south of England. They found that floods are linked with an earache, psychological distress, and gastroenteritis. Psychological damage may illustrate some of the additional physical illnesses recorded by affected people, and likely even by children. Strategies to encourage the adaptation of the community to disasters, where flood management has deteriorated will provide logistical assistance for flood victims and adequate therapeutic help. However, in Bangladesh, studies conducted on the causes and spread of diarrhea at the expense of flooding. It highlighted the important demographic, economic, and social aspects from the preview of amenities provided by the system and how the provision of food and clean drinking water becomes essential during and after flooding ( Kunii et al., 2002 ).
The prevalence of diarrheal disease and its associated epidemiological factors were examined by ( Mondal et al., 2001 ), by applying a systematic random sampling analysis on the data of two identified flood-prone regions in West Bengal’s Midnapur area. The research found the diarrheal disease to be the most severe morbidity in flood-prone communities. Some habits, such as using pond water for utensil washing and cooking purposes, hand washing after soap-less defecation, inadequate washing hands before feeding, open area defecation, storing of drinkable water in large mouth containers, etc. , were found to be correlated with increased diarrhea attack levels, both in research and control community during flooding to the pre-flooded period ( Mondal et al., 2001 ).
Access to medical care and drugs is of central concern in the flood-affected areas of Pakistan, as is the reconstruction of community health facilities in the region. According to the WHO, 2010s flood affected more than 20 million people, many of whom were homeless. At that time, at least 8 million people required urgent humanitarian aid. More than 400 of the approximately 3000 clinics and hospitals in flood-affected areas had been affected or closed, thereby restricting the availability of urgent and daily healthcare. The availability to clean water for drinking and standards of health and safety were severely affected, though the risks of occurrences of waterborne diseases. Specific health issues, such as tuberculosis , skin diseases, severe respiratory diseases, and starvation, were of utmost concern ( WHO, 2010 ).
3.3 Economic cost of floods
The future of the world economy is more uncertain than it has ever been, and this uncertainty is sensitive to uncertainties relating to a range of economic policy decisions made by all parties involved, including governments ( Işık et al., 2019 ). Natural climatic risks have direct interaction with core macroeconomic factors and can affect economic development and market performance rapidly. Jonkman et al. (2008) described a framework established for the assessment of flood damage in the Netherlands and suggested that the economic loss resulting from the floods relies on the country’s vulnerable location, coastal zone, and economy. According to ( Toya and Skidmore, 2007 ), high-income economies, high schooling, more transparency, more robust financial structures, and reduced government suffer fewer casualties from natural disasters. They argue that private preference for safety nets rises because of increasing people’s wages because higher salaries encourage individuals to mitigate the danger by investing extra in precautionary measures.
With improved GDP growth, citizens could have improved facilities, alarming networks, and flood-prone precautionary and protective steps that can mitigate the effect of floods. Sadia et al. (2013) examined the impact of natural disaster deaths on Pakistan’s GDP per capita from the disaster by taking the data for the period 1975 to 2009, using the ordinary least square model, and found a strong positive impact on per capita GDP from disaster-related deaths, human capital, and life expectancy.
Ahmad et al. (2011) proposed that two levels of flood damage could be evaluated. The harm to facilities (infrastructure) and mortality can be called the first tragedy accompanied by second disasters like the families facing poverty due to the death of earning hands. The risk of the second catastrophe may be higher than that of the first. The risk of natural hazards along with people’s socio-economic insecurity presents a major threat to the system of Pakistan’s government.
Sardar et al. (2016) discuss three threats linked to floods: death, property destruction, and non-fatal community consequences, and calculated the effect of these disasters on Pakistan’s GDP growth for the duration 1972–2013. Their results showed that the per capita GDP development and emergency prevention reduce the severity of flood risks associated with it. Most notably, and contrary to evidence from several nations, flood intensity accentuates flood-related hazards in Pakistan which indicate a lack of understanding of previous flood experience. Concerning the flood-to-economic growth connection, their analysis showed that flood-related threats have a substantial negative effect on the economy’s GDP performance. Property loss causes economic development with the greatest effects.
3.4 Housing
According to the economic survey of Pakistan in 2011, approximately 392,786 houses were affected, and 728,192 were lost. In the districts of Muzaffargarh and Rajanpur in Punjab, Nowshera, and D. I. Khan in KPK and Jaffarabad, Jacobabad, Shikarpur, and Thatta in Sindh, the harm was more noticeable also shown in Figure 1 and Table 1 . The geographical distribution of Pakistan’s 2010 floods direct and indirect showed in Figure 3 and Figure 4 , respectively. The flood damages and reconstruction costs by sector as shown in Table 4 .
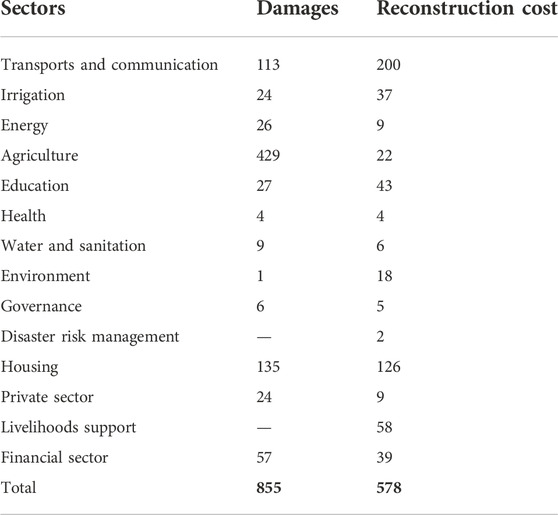
TABLE 4 . Flood damages and reconstruction cost by sectors (Rs. in Billion) ( Pakistan: Flood Impact Assessment, 2011 ).
4 Flood management in Pakistan
The flood prevention strategy is a relatively complicated problem in Pakistan. In each of the four provinces, the complexity of the issue differs because of their specific physiographic, climatic, geographical, and socioeconomic circumstances ( Chaudhri, 1981 ). Early severe flooding happened in 1950, 1956, and 1957 after independence. However, no systematic flood control program was implemented at the national level, owing to scarce funding and administrative structures. Protection and control of flooding remained the exclusive responsibility of regional governments until 1976. That improved after 1973 destroying floods that took 474 lives and caused damage of 160 billion Pakistani Rupees ( Tariq and van de Giesen, 2012 ).
4.1 Evolution of flood management system in Pakistan
In 1973 Pakistan faced severe floods which led to the establishment of the Federal Flood Commission (FFC) in 1977. The commission worked under the Ministry of Water and Power and was established to implement nationwide flood management, particularly concerning the Indus River Basin. The main functions of the FFC include developing national flood management measures; approving flood management plans drawn up by local governments and federal entities; examining flood damage to facilities in the public sector, and analysis of repair and rehabilitation plans; flood forecasting, and alert program enhancement measures; providing guidelines on standards for the management of flood protection reservoirs ( GoP Annual Flood Report, 2009 ).
The first National Flood Protection Plan (NFPP-I) was developed after the creation of the FFC, with a spending timeline to be introduced throughout the 1978–1988 decade. A Federal Coordination Cell (now reshaped as FID Cell) was formed in 1982 to organize the Provincial Irrigation Departments ' operations, especially in the drainage region. In 1987 Dam Protection Council was set up to examine established dams via DSO WAPDA and proposals for new dams etc. ( GoP Annual Flood Report, 2009 ).
In Pakistan, flood control initiatives consist primarily of flood-protection embankments, spurs, studs, and sophisticated flood-prediction strategies. The provincial governments have developed numerous flood-protection systems to address local flood challenges ( Baig, 2008 ). According to Ahmed et al. (2014) flood risk reduction in Pakistan was handled primarily by federal and provincial authorities, which typically need to be reconsidered to find innovative methods and strategies to counter the threat. Flood management institutions and their responsibilities are presented in Figure 8 .

FIGURE 8 . Evolution of flood management and relief system in Pakistan.
4.2 Flood warning system in Pakistan
According to Jain et al. (2018) , Flood Forecasting and Warning System (FFWS) main aim is to inform the public and other stakeholders of an imminent flood as early and effectively as possible. In Pakistan, the mechanism of flood warning and control undertakes three phases: 1) The PMD tracks the monsoon weather pattern, which produces either from the west in the Arabian Sea or from the East in the Bay of Bengal, in the first stage. Their movements are monitored for the upper catchments lying in Pakistan or over the border, and estimates are generated one to 2 days in advance for expected rains and the severity of such rains. In the case of rains, the volume of rainfall is estimated and evaluated above the rim stations for their possible run-off relationship) after that is the stage of flood creation that starts with the generation of runoff from the rim stations and flows down into the Indus River and its tributary. Projected rainfall and flow data and real upstream flows are the hydro-meteorological portions of the flood prediction method 3) ultimately, the hydrological part of the forecasting network is to track and control the route of the flood wave below the rim station of the rivers at the downstream locations. This is handled by WAPDA and controlled in respective provinces by the irrigation departments ( Hussain, 2015 ).
The early warning system of Pakistan is efficient to some extent; however, the flood warning system only aims to provide information, rather protective measures. In our opinion, as we have also mentioned in the study, such acts can only help lessen the losses to be incurred. However, the implementation in the form of evacuation, protection, and migration is based upon the behavioral choices of the people of the area. We can mention the example of Mardan, where legal force was used to vacate the city during the 2022 floods. Thus, flood warning system calculates and provides relevant information. However, the impact must reach the grassroots level, furthermore, there is a strong need of creating a civic sense among the people of Pakistan. As can be seen in the case of Sawat River flash floods, it is speculated that most of the socio-economic loss incurred was caused by illegitimate activities, land grabbing on the riverbed, and around the torrent flow, which was against the River Protection Ordinance, 2002.
4.2.1 Flood forecasting division’s flood forecasting models
The FFD employs the Flood Early Warning System (FEWS) and the Indus Integrated Flood Analysis System (IFAS) for flood forecasts. In terms of urban areas, flood prediction and warning systems were developed in the Nallah Lai Basin ( Afsa et al., 2013 ; Sugiura et al., 2014 ). Since 2007 the FFD has used the flood forecasting framework FEWS built by Delft Hydraulic in the Netherlands. The FEWS contains (1) the rainfall-runoff interface Sacramento Soil Moisture Accounting (SAC-SMA) and (2) the SOBEK, an interface for hydraulic fluid routing ( Shrestha et al., 2019 ). The Layout contains data from forty-four (44) WAPDA telemetry stations. The rainfall-runoff process performance determines the entrance into the routing mechanism ( Awan, 2003 ). Early warning mechanism components are shown in Figure 9 .
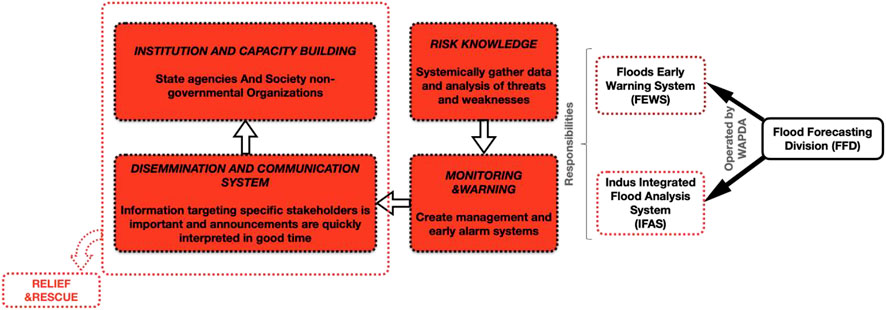
FIGURE 9 . Early warning mechanism components ( Hussain, 2015 ).
From the perspective of South Asia, the hierarchical institutional structure Flash Flood Guidance System with Global Coverage (FFGS) can provide warnings about 6–24 h in advance for South Asian countries, including India, Nepal, Bhutan, Bangladesh, and Sri Lanka. However, the respective NDMA’s of these countries perform the same functions as Pakistan, Since Bangladesh and India are more densely populated than Pakistan, the riverbank protection in India and Bangladesh is much more rooted at the state level, and powers are transferred to the grassroots level for implementation. But, as the cultural roots of the subcontinent remain the same along with legal roots, the implementation and behavioral intention part remain at certain risk.
4.2.1.1 Criticism
The present flood forecasting method of the FEWS model, however, has minimal regional scope, and rainfall-runoff knowledge and downstream routing of the Tarbela and Kabul rivers are not integrated with the model. The tributaries included in the FEWS are not determined by the amount and rate of discharge necessary for reliable flood forecasting ( Shrestha et al., 2019 ). The FFD also introduces the IFAS established by the International Center for Water Hazard and Risk Management (ICHARM) under the Critical Reinforcing of Pakistan’s Flood Warning and Management Capacity Program and the Japan Aerospace Exploration Agency (JAXA), with UNESCO funding. More than thirty-nine (39) districts in Pakistan are protected by flood prediction and early warning services from the IFAS ( Ahmad, 2015 ; Mustafa et al., 2015 ).
Disaster management institutions in Pakistan are relatively new and need time to strengthen their foothold. National disaster management authority (NDMA) claims a preparedness-oriented approach, but at the core, the country’s disaster management system is still operating on a top-down approach. Practically, it is more relief-focused, and season-based planning is being implemented. Under the 18th amendment of the constitution of Pakistan, PDMAs are not legally bound to follow NDMA. As a result, NDMA just acts as a general policy-defining institution. Mutual coordination needs to be encouraged to cover potential oversights. District disaster management authorities are composed of officials from different local institutions and are virtually non-existent in the field. Thus, a dedicated DDMA is needed, which could act as a catalyst in disaster risk reduction ( Rana et al., 2021 ).
There is also a need for regular technical and institutional capacity assessments for effective disaster preparedness and response. Consequently, ad-hoc disaster risk management is happening in the study areas. More research could be conducted to identify potential weaknesses in these urban institutions. There is a need to improve local institutions’ image in front of communities as strong distrust further complicates flood risk reduction initiatives. Local institutions and communities need to work together to realize the full potential of the disaster management cycle and disaster risk reduction approach ( Rana et al., 2021 ). Flood management institutions and their responsibilities are shown in Figure 10 .
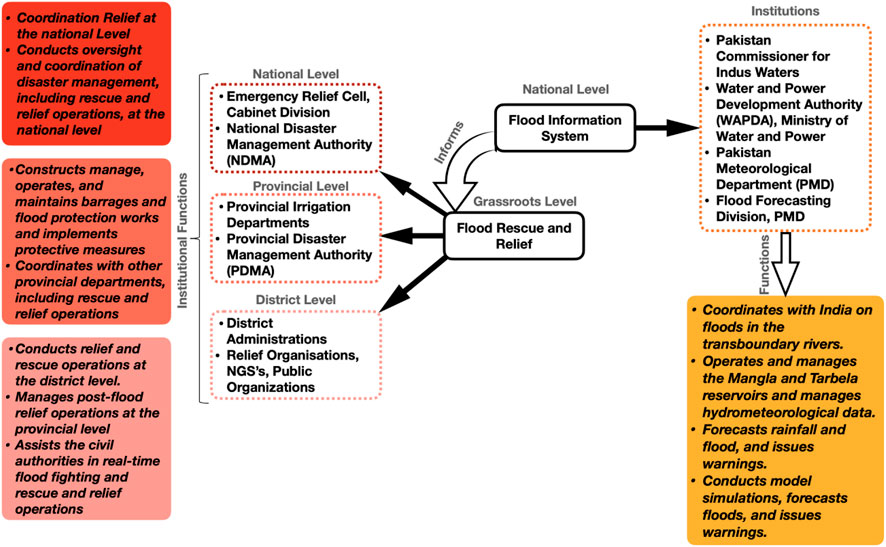
FIGURE 10 . Flood management institutions and their responsibilities developed after ( Ali, 2013 ; Rana et al., 2021 ).
To cope with the situation, there must be a multi-pronged approach, as suggested there is a strong need for water reservoirs, which can be fulfilled through water policy. Secondly, the early warning system and relevant institutions must reach the grassroots level to cope with the emergency in a better way. Thirdly, the whole management machinery of the area remains dependent upon a certain institution, Pakistan Administrative Services. There is a strong to segregate the departmental responsibilities and develop new departments ensuring implementation. Lastly, the citizens must have a civic sense or understanding of the nature of the information provided to them in the wake of floods.
In April 2018, Pakistan’s four provincial chief ministers endorsed the country’s first regional water policy. The National Water Policy’s goal is to address the growing water problem and include an overarching policy structure and recommendations for a robust action plan. The National Water Policy provides a specific policy structure and collection of water protection standards based on which the Provincial Governments will devise their development plans and water conservation, water improvement, and water management projects ( GoP National Water Policy, 2018 ).
5 Conclusion
Floods are a frequently occurring phenomenon due to heavy monsoon rains in Pakistan. The size and magnitude of the floods that Pakistan has confronted in the last few years would have been a problem for any nation. Recent severe floods have shown that there is a lack of adequate cooperation between flood control agencies, due in part to shortcomings in current technological capacities, such as warning signals, preparedness initiatives, disaster response, and systemic flood prevention measures. This is important to further develop flood monitoring and alert systems to reduce the damages of potential floods. Although Pakistan’s flood warning and detection systems have shown their effectiveness, the forecasting ability of the network is still weak. At the same time the institutions, NDMA and PDMA, operate at national and provincial levels, dispersing the rehabilitation responsibilities to local bureaucracy rather than establishing a grassroots-level structure with reference to small cities and villages. It also makes rehabilitation procedures weak and ineffective in most areas. Nonetheless, a cohesive response by the Pakistani community and the combined efforts of all the international and domestic agencies concerned remained crucial. Only providing damage reimbursements to flood victims is not the remedy; we need to get rid of this problem. Pakistan’s water management also needs to develop more reservoirs—lakes, and dams as a way to combat floods. The government should generate and incorporate robust public awareness initiatives to educate the public on flood risks and flood preparedness. In conclusion, to achieve effective flood control, a risk-based proactive strategy is needed.
Author contributions
All authors listed have made a substantial, direct, and intellectual contribution to the work and approved it for publication.
Open access funding provided by UiT The Arctic University of Norway.
Conflict of interest
The authors declare that the research was conducted in the absence of any commercial or financial relationships that could be construed as a potential conflict of interest.
Publisher’s note
All claims expressed in this article are solely those of the authors and do not necessarily represent those of their affiliated organizations, or those of the publisher, the editors and the reviewers. Any product that may be evaluated in this article, or claim that may be made by its manufacturer, is not guaranteed or endorsed by the publisher.
Afsa, M. M., Aslam, K., and Atif, S. “Comparative analysis of flood inu by flood early warning system 2010 floods in Pakistan,” in 5th World Engineering Congress , Islamabad, Pakistan , 2013.
Google Scholar
Ahern, M., Kovats, R. S., Wilkinson, P., Few, R., and Matthies, F. (2005). Global health impacts of floods: Epidemiologic evidence. Epidemiol. Rev. 27, 36–46. doi:10.1093/epirev/mxi004
PubMed Abstract | CrossRef Full Text | Google Scholar
Ahmad, F., Kazmi, S. F., and Pervez, T. (2011). Human response to hydro-meteorological disasters: A case study of the 2010 flash floods in Pakistan. J. Geogr. Regional Plan. 4 (9), 518.
Ahmad, N. (2015). Early warning systems and Disaster risk information. LEAD , 1–2.
Ahmad, N., Khan, S., Ehsan, M., Rehman, F. U., and Al-Shuhail, A. (2022). Estimating the total volume of running water bodies using geographic information system (gis): A case study of peshawar basin (Pakistan). Sustainability 14 (7), 3754. doi:10.3390/su14073754
CrossRef Full Text | Google Scholar
Ahmed, B., Wei, S., Fu, Y. G., Shabbir, M., and Nabi, G. (2014). Effects of floods policy in Pakistan and management issues:(case of district dera-ghazi khan). Int. J. Adv. Res. 2 (10), 967–974.
Ali, A. (2013). Indus Basin floods mechanisms, impacts, and management . Philippines: Asian Development Bank , 67.
Ali, S., Liu, Y., Ishaq, M., Shah, T., Ilyas, A., Din, I. U., et al. (2017). Climate change and its impact on the yield of major food crops: Evidence from Pakistan. Foods 6 (6), 39. doi:10.3390/foods6060039
Allaire, M. (2018). Socio-economic impacts of flooding: A review of the empirical literature. Water Secur. 3, 18–26. doi:10.1016/j.wasec.2018.09.002
Anbarci, N., Escaleras, M., and Register, C. A. (2005). Earthquake fatalities: The interaction of nature and political economy. J. Public Econ. 89 (9-10), 1907–1933. doi:10.1016/j.jpubeco.2004.08.002
Ashraf, A., Iqbal, M. B., Mustafa, N., Naz, R., and Ahmad, B. (2021). Prevalent risk of glacial lake outburst flood hazard in the Hindu Kush–Karakoram–Himalaya region of Pakistan. Environ. Earth Sci. 80 (12), 1–12. doi:10.1007/s12665-021-09740-1
Ashraf, A., Naz, R., and Roohi, R. (2012). Glacial lake outburst flood hazards in Hindukush, karakoram and himalayan ranges of Pakistan: Implications and risk analysis. Geomatics, Nat. Hazards Risk 3 (2), 113–132. doi:10.1080/19475705.2011.615344
Ashraf, S., Iftikhar, M., Shahbaz, B., Khan, G. A., and Luqman, M. (2013). Impacts of flood on livelihoods and food security of rural communities: A case study of southern Punjab, Pakistan. Pak. J. Agric. Sci. 50 (4), 751–758.
Asian Development Bank (2010). Pakistan floods 2010 preliminary damage and needs assessment . Islamabad, Pakistan: Asian Development Bank , 188.
Awan, S. A. (2003). In Flood forecasting and management in Pakistan, IUGG, Sapporo . Sapporo: IAHS Publ , 90–98.
Baig, M. (2008). In Floods and flood plains in Pakistan . Lahore, Pakistan: ICID .
Bukhari, S., and Rizvi, S. (2017). Impact of 2010 floods on Pakistan’s Agriculture. J. Environ. Anal. Toxicol. 7 (1), 1–4. doi:10.4172/2161-0525.1000424
Carrivick, J. L., and Tweed, F. S. (2016). A global assessment of the societal impacts of glacier outburst floods. Glob. Planet. Change 144, 1–16. doi:10.1016/j.gloplacha.2016.07.001
Chaudhri, S. A. (1981). In flood characteristics and problems in Pakistan, natural resources forum . Hoboken, New Jersey: Wiley Online Library , 399–407.
Chaudhry, Q. (2017). Climate change profile of Pakistan . Mandaluyong, Philippines: Asian development bank .
Commission, F. F. (2007). Annual flood report . Islamabad, Pakistan: Ministry of Water and Power Pakistan and Federal Flood Commission .
Deen, S. (2015). Pakistan 2010 floods Policy gaps in disaster preparedness and response. Int. J. Disaster Risk Reduct. 12, 341–349. doi:10.1016/j.ijdrr.2015.03.007
Du, W., FitzGerald, G. J., Clark, M., and Hou, X.-Y. (2010). Health impacts of floods. Prehosp. Disaster Med. 25 (3), 265–272. doi:10.1017/s1049023x00008141
GoP Annual Flood Report (2009). Federal flood commission . Pakistan: Islamabad, Pakistan: Ministry of Water & Power , 44.
GoP annual flood report; Federal flood commission , Ministry of Water Resources , Pakistan: Islamabad. Pakistan , 2017; p 118.
GoP Economic Survey (2011). GoP economic survey 2010-11 . Islamabad, Pakistan: Government of Pakistan , 14.
GoP National Water Policy (2018). GoP national water policy . Pakistan: Ministry of Water Resources, Government of Pakistan Islamabad .
Gop Pakistan Economic Survey (2019). GoP Pakistan economic survey 2018-19 . Islamabad, Pakistan: Government of Pakistan Finance Division .
GoP, W. (2011). Disease early warning system and response in Pakistan. Wkly. Epidemiol. Bull. 2 (29), 1–8.
Hussain, S. S. (2015). Pakistan 2010 floods: Causes and lessons learnt . Pakistan: Islamabad, Pakistan: Oxfam .
IFRC (2020). South Asia floods: 9.6 million people swamped as humanitarian crisis deepens . Kuala Lumpur/Delhi/Dhaka/Kathmandu/Geneva: IFRC .
Iqbal, S., Khan, A. N., Jadoon, M. A., and Alam, I. (2018). Effects of flood-2010 on agricultural sector in khyber Pakhtunkhwa: A case of district charsadda. Sarhad J. Agric. 34 (1), 215–219. doi:10.17582/journal.sja/2018/34.1.215.219
Işık, C., Ahmad, M., Ongan, S., Ozdemir, D., Irfan, M., and Alvarado, R. (2021). Convergence analysis of the ecological footprint: Theory and empirical evidence from the USMCA countries. Environ. Sci. Pollut. Res. 28 (25), 32648–32659. doi:10.1007/s11356-021-12993-9
Işık, C., Ongan, S., Bulut, U., Karakaya, S., Irfan, M., Alvarado, R., et al. (2022). Reinvestigating the Environmental Kuznets Curve (EKC) hypothesis by a composite model constructed on the Armey curve hypothesis with government spending for the US States. Environ. Sci. Pollut. Res. 29 (11), 16472–16483. doi:10.1007/s11356-021-16720-2
Isik, C., Ongan, S., Ozdemir, D., Ahmad, M., Irfan, M., Alvarado, R., et al. (2021). The increases and decreases of the environment Kuznets curve (EKC) for 8 OECD countries. Environ. Sci. Pollut. Res. 28 (22), 28535–28543. doi:10.1007/s11356-021-12637-y
Işık, C., Sirakaya-Turk, E., and Ongan, S. (2019). Testing the efficacy of the economic policy uncertainty index on tourism demand in USMCA: Theory and evidence. Tour. Econ. 26 (8), 1344–1357. doi:10.1177/1354816619888346
Jain, S. K., Mani, P., Jain, S. K., Prakash, P., Singh, V. P., Tullos, D., et al. (2018). A Brief review of flood forecasting techniques and their applications. Int. J. River Basin Manag. 16 (3), 329–344. doi:10.1080/15715124.2017.1411920
Jonkman, S. N., Bočkarjova, M., Kok, M., and Bernardini, P. (2008). Integrated hydrodynamic and economic modelling of flood damage in The Netherlands. Ecol. Econ. 66 (1), 77–90. doi:10.1016/j.ecolecon.2007.12.022
Kahn, M. E. (2005). The death toll from natural disasters: The role of income, geography, and institutions. Rev. Econ. Statistics 87 (2), 271–284. doi:10.1162/0034653053970339
Kirigia, J. M., Sambo, L. G., Aldis, W., and Mwabu, G. M. (2004). Impact of disaster-related mortality on gross domestic product in the WHO African Region. BMC Emerg. Med. 4 (1), 1–9. doi:10.1186/1471-227x-4-1
Kondo, H., Seo, N., Yasuda, T., Hasizume, M., Koido, Y., Ninomiya, N., et al. (2002). Post-flood—Infectious diseases in Mozambique. Prehosp. Disaster Med. 17 (3), 126–133. doi:10.1017/s1049023x00000340
Kreft, S., Eckstein, D., Junghans, L., Kerestan, C., and Hagen, U. (2015). Global climate risk index 2015: Who suffers most from extreme weather events? Weather-related loss events in 2013 and 1994 to 2013 . Bonn, Germany: Germanwatche.V , 1–32.
Kronstadt, K. A. (2010). Flooding in Pakistan: Overview and issues for congress . Collingdale, United States: DIANE Publishing .
Kunii, O., Nakamura, S., Abdur, R., and Wakai, S. (2002). The impact on health and risk factors of the diarrhoea epidemics in the 1998 Bangladesh floods. Public Health 116 (2), 68–74. doi:10.1016/s0033-3506(02)00506-1
McMullen, L. E., and Lytle, D. A. (2012). Quantifying invertebrate resistance to floods: A global-scale meta-analysis. Ecol. Appl. 22 (8), 2164–2175. doi:10.1890/11-1650.1
Mohanty, L., and Maiti, S. (2021). Probability of glacial lake outburst flooding in the Himalaya. Resour. Environ. Sustain. 5, 100031. doi:10.1016/j.resenv.2021.100031
Mondal, N., Biswas, R., and Manna, A. (2001). Risk factors of diarrhoea among flood victims: A controlled epidemiological study. Indian J. Public Health 45 (4), 122–127.
PubMed Abstract | Google Scholar
Morgan, O., Ahern, M., and Cairncross, S. (2005). Revisiting the tsunami: Health consequences of flooding. PLoS Med. 2 (6), e184–e493. doi:10.1371/journal.pmed.0020184
Morris, J., and Brewin, P. (2014). The impact of seasonal flooding on agriculture: The spring 2012 floods in somerset, england. J. Flood Risk Manag. 7 (2), 128–140. doi:10.1111/jfr3.12041
Mustafa, D., Gioli, G., Qazi, S., Waraich, R., Rehman, A., and Zahoor, R. (2015). Gendering flood early warning systems: The case of Pakistan. Environ. Hazards 14 (4), 312–328. doi:10.1080/17477891.2015.1075859
NDMA (2019). National disaster response plan 2019 (NDRP-2019) . Islamabad, Pakistan: NDMA, Government of Pakistan .
Nie, Y., Sheng, Y., Liu, Q., Liu, L., Liu, S., Zhang, Y., et al. (2017). A regional-scale assessment of himalayan glacial lake changes using satellite observations from 1990 to 2015. Remote Sens. Environ. 189, 1–13. doi:10.1016/j.rse.2016.11.008
Pakistan: Flood Impact Assessment (2011). GoP an economic survey of Pakistan 2010-2011’s special section . Islamabad, Pakistan: Pakistan: Flood Impact Assessment , 2.
Parida, Y. (2019). Economic impact of floods in the Indian states. Environ. Dev. Econ. 25, 267–290. doi:10.1017/s1355770x19000317
Rafiq, L., and Blaschke, T. (2012). Disaster risk and vulnerability in Pakistan at a district level. Geomatics, Nat. Hazards Risk 3 (4), 324–341. doi:10.1080/19475705.2011.626083
Rana, I. A., Asim, M., Aslam, A. B., and Jamshed, A. (2021). Disaster management cycle and its application for flood risk reduction in urban areas of Pakistan. Urban Clim. 38, 100893. doi:10.1016/j.uclim.2021.100893
Reacher, M., McKenzie, K., Lane, C., Nichols, T., Kedge, I., Iversen, A., et al. (2004). Health impacts of flooding in Lewes: A comparison of reported gastrointestinal and other illness and mental health in flooded and non-flooded households. Commun. Dis. Public Health 7 (1), 39–46.
Rehman, A., Jingdong, L., Du, Y., Khatoon, R., Wagan, S. A., and Nisar, S. K. (2015). Flood disaster in Pakistan and its impact on agriculture growth (a review). Glob. Adv. Res. J. Agric. Sci. 4 (12), 827–830.
Rehman, H., and Kamal, A. “In Indus Basin river system-Flooding and flood mitigation,” in 8th International River Symposium , Brisbane, Australia , 2-11 September 2005 (Brisbane: IISD ), 2–11.
Rufat, S., Tate, E., Burton, C. G., and Maroof, A. S. (2015). Social vulnerability to floods: Review of case studies and implications for measurement. Int. J. disaster risk Reduct. 14, 470–486. doi:10.1016/j.ijdrr.2015.09.013
Sadia, B., Bashir, M. A., Nawaz, M., and Zaman, K. (2013). Effects of disaster-related mortality on gross domestic product in Pakistan. Int. J. Ecol. Dev. 24 (1), 62–80.
Sardar, A., Javed, S. A., and Amir-ud-Din, R. (2016). Natural disasters and economic growth in Pakistan: An enquiry into the floods related hazards’ triad . Islamabad: Pakistan Institute of Development Economics .
Shafi, A., Wang, Z., Ehsan, M., Riaz, F. A., Ali, M. R., Mamodson, Z. A., et al. (2022). A game theory approach to the logic of illegitimate behavior induced during land conflict litigation in urban and peri-urban areas of Pakistan. Cities 130, 103990. doi:10.1016/j.cities.2022.103990
Shrestha, M. S., Khan, M. R., Wagle, N., Babar, Z. A., Khadgi, V. R., and Sultan, S. (2019). “Chapter 13 - review of hydrometeorological monitoring and forecasting system for floods in the Indus Basin in Pakistan,” in Indus River basin . Editors S. I. Khan, and T. E. Adams (Amsterdam, Netherlands: Elsevier ), 309–333.
Sugiura, A., Fujioka, S., Nabesaka, S., Sayama, T., Iwami, Y., Fukami, K., et al. (2014). Challenges on modelling a large River Basin with scarce data: A case study of the Indus upper catchment. J. Hydrology Environ. Res. 2 (1), 59–64.
Tariq, M., and van de Giesen, N. (2012). Floods and flood management in Pakistan. Phys. Chem. Earth, Parts A/B/C 47-48, 11–20. doi:10.1016/j.pce.2011.08.014
Toya, H., and Skidmore, M. (2007). Economic development and the impacts of natural disasters. Econ. Lett. 94 (1), 20–25. doi:10.1016/j.econlet.2006.06.020
WHO WHO Pakistan: The health impact of the floods. Available at: https://www.who.int/hac/crises/pak/highlights/september2010/en/ . (Accessed December 25, 2021)
Yaqub, M., Eren, B., and Doğan, E. (2015). Flood causes, consequences and protection measures in Pakistan. Disaster Sci. Eng. 1 (1), 8–16.
Keywords: major floods, flood management, social and economic cost, flood control, flood impacts
Citation: Manzoor Z, Ehsan M, Khan MB, Manzoor A, Akhter MM, Sohail MT, Hussain A, Shafi A, Abu-Alam T and Abioui M (2022) Floods and flood management and its socio-economic impact on Pakistan: A review of the empirical literature. Front. Environ. Sci. 10:1021862. doi: 10.3389/fenvs.2022.1021862
Received: 17 August 2022; Accepted: 22 November 2022; Published: 01 December 2022.
Reviewed by:
Copyright © 2022 Manzoor, Ehsan, Khan, Manzoor, Akhter, Sohail, Hussain, Shafi, Abu-Alam and Abioui. This is an open-access article distributed under the terms of the Creative Commons Attribution License (CC BY). The use, distribution or reproduction in other forums is permitted, provided the original author(s) and the copyright owner(s) are credited and that the original publication in this journal is cited, in accordance with accepted academic practice. No use, distribution or reproduction is permitted which does not comply with these terms.
*Correspondence: Muhsan Ehsan, [email protected] ; Tamer Abu-Alam, [email protected]
This article is part of the Research Topic
Eco-Innovation and Green Productivity for Sustainable Production and Consumption
Thank you for visiting nature.com. You are using a browser version with limited support for CSS. To obtain the best experience, we recommend you use a more up to date browser (or turn off compatibility mode in Internet Explorer). In the meantime, to ensure continued support, we are displaying the site without styles and JavaScript.
- View all journals
- Explore content
- About the journal
- Publish with us
- Sign up for alerts
- Published: 04 October 2023
Global evidence of rapid urban growth in flood zones since 1985
- Jun Rentschler ORCID: orcid.org/0000-0002-2014-2124 1 ,
- Paolo Avner ORCID: orcid.org/0000-0002-0153-5205 1 ,
- Mattia Marconcini ORCID: orcid.org/0000-0002-5042-5176 2 , 3 ,
- Emanuele Strano 3 ,
- Michalis Vousdoukas 4 &
- Stéphane Hallegatte ORCID: orcid.org/0000-0002-1781-4268 1
Nature volume 622 , pages 87–92 ( 2023 ) Cite this article
15k Accesses
19 Citations
578 Altmetric
Metrics details
- Natural hazards
Disaster losses are increasing and evidence is mounting that climate change is driving up the probability of extreme natural shocks 1 , 2 , 3 . Yet it has also proved politically expedient to invoke climate change as an exogenous force that supposedly places disasters beyond the influence of local and national authorities 4 , 5 . However, locally determined patterns of urbanization and spatial development are key factors to the exposure and vulnerability of people to climatic shocks 6 . Using high-resolution annual data, this study shows that, since 1985, human settlements around the world—from villages to megacities—have expanded continuously and rapidly into present-day flood zones. In many regions, growth in the most hazardous flood zones is outpacing growth in non-exposed zones by a large margin, particularly in East Asia, where high-hazard settlements have expanded 60% faster than flood-safe settlements. These results provide systematic evidence of a divergence in the exposure of countries to flood hazards. Instead of adapting their exposure, many countries continue to actively amplify their exposure to increasingly frequent climatic shocks.
This is a preview of subscription content, access via your institution
Access options
Access Nature and 54 other Nature Portfolio journals
Get Nature+, our best-value online-access subscription
24,99 € / 30 days
cancel any time
Subscribe to this journal
Receive 51 print issues and online access
185,98 € per year
only 3,65 € per issue
Rent or buy this article
Prices vary by article type
Prices may be subject to local taxes which are calculated during checkout
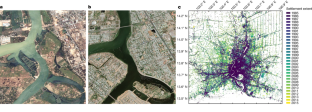
Similar content being viewed by others
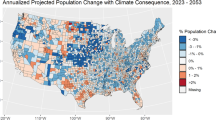
Integrating climate change induced flood risk into future population projections
Evelyn G. Shu, Jeremy R. Porter, … Edward Kearns
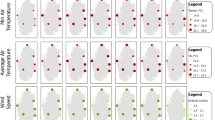
Exploring urban growth–climate change–flood risk nexus in fast growing cities
Salah Basem Ajjur & Sami G. Al-Ghamdi
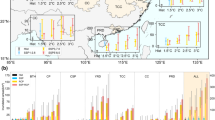
Substantial increase in future fluvial flood risk projected in China’s major urban agglomerations
Ruijie Jiang, Hui Lu, … Fuqiang Tian
Data availability
The WSF-Evo dataset is publicly available for download from https://geoservice.dlr.de/web/maps/eoc:wsfevolution . We use proprietary global fluvial and pluvial flood-hazard data with the permission of Fathom Global, who provide the data for academic purposes and can be contacted at https://www.fathom.global/contact-us/ . We use coastal flood maps developed by Vousdoukas et al. 40 , which are publicly available for download from https://doi.org/10.5281/zenodo.8057902 . Country-level summary results and subnationally and annually disaggregated results are provided in the Supplementary Information to this study.
Code availability
The source code for this study is available at https://doi.org/10.5281/zenodo.7987230 .
Schiermeier, Q. Droughts, heatwaves and floods: how to tell when climate change is to blame. Nature 560 , 20–22 (2018).
Article ADS CAS PubMed Google Scholar
Otto, F. et al. The attribution question. Nat. Clim. Change 6 , 813–816 (2016).
Article ADS Google Scholar
Philip, S. et al. A protocol for probabilistic extreme event attribution analyses. Adv. Stat. Climatol. Meteorol. Oceanogr. 6 , 177–203 (2020).
Raju, E., Boyd, E. & Otto, F. Stop blaming the climate for disasters. Commun. Earth Environ. 3 , 1 (2022).
Lahsen, M. & Ribot, J. Politics of attributing extreme events and disasters to climate change. Wiley Interdiscip. Rev. Clim. Change 13 , e750 (2022).
Article Google Scholar
Hallegatte, S., Vogt-Schilb, A., Bangalore, M. & Rozenberg, J. Unbreakable: Building the Resilience of the Poor in the Face of Natural Disasters (World Bank, 2017).
Tellman, B. et al. Satellite imaging reveals increased proportion of population exposed to floods. Nature 596 , 80–86 (2021).
Henderson, J. V. Cities and development. J. Reg. Sci. 50 , 515–540 (2010).
Article PubMed PubMed Central Google Scholar
Rosenthal, S. S. & Strange, W. C. in Handbook of Regional and Urban Economics Vol. 4 (eds Henderson, J. V. & Thisse, J.-F.) 2119–2171 (Elsevier, 2004).
Duranton, G. & Puga, D. in Handbook of Regional and Urban Economics Vol. 4 (eds Henderson, J. V. & Thisse, J.-F.) 2063–2117 (2004).
Neumann, B., Vafeidis, A. T., Zimmermann, J. & Nicholls, R. J. Future coastal population growth and exposure to sea-level rise and coastal flooding - a global assessment. PLoS One 10 , e0118571 (2015).
Peduzzi, P., Dao, H., Herold, C. & Mouton, F. Assessing global exposure and vulnerability towards natural hazards: the Disaster Risk Index. Nat. Hazards Earth Syst. Sci. 9 , 1149–1159 (2009).
de Bruijn, J. A. et al. A global database of historic and real-time flood events based on social media. Sci. Data 6 , 311 (2019).
Muis, S., Verlaan, M., Winsemius, H. C., Aerts, J. C. & Ward, P. J. A global reanalysis of storm surges and extreme sea levels. Nat. Commun. 7 , 11969 (2016).
Article ADS CAS PubMed PubMed Central Google Scholar
Rentschler, J., Salhab, M. & Jafino, B. A. Flood exposure and poverty in 188 countries. Nat. Commun. 13 , 3527 (2022).
Arnell, N. W. & Gosling, S. N. The impacts of climate change on river flood risk at the global scale. Clim. Change 134 , 387–401 (2016).
Dottori, F. et al. Increased human and economic losses from river flooding with anthropogenic warming. Nat. Clim. Change 8 , 781–786 (2018).
Andreadis, K. et al. Urbanizing the floodplain: global changes of imperviousness in flood-prone areas. Environ. Res. Lett. 17 , 104024 (2022).
Smith, A. et al. New estimates of flood exposure in developing countries using high-resolution population data. Nat. Commun. 10 , 1814 (2019).
Article ADS PubMed PubMed Central Google Scholar
Kocornik-Mina, A., McDermott, T. K. J., Michaels, G. & Rauch, F. Flooded cities. Am. Econ. J. Appl. Econ. 12 , 35–66 (2020).
Winsemius, H. et al. Global drivers of future river flood risk. Nat. Clim. Change 6 , 381–385 (2016).
Hirabayashi, Y. et al. Global flood risk under climate change. Nat. Clim. Change 3 , 816–821 (2013).
Jongman, B., Ward, P. & Aerts, J. Global exposure to river and coastal flooding: long term trends and changes. Glob. Environ. Change 22 , 823–835 (2012).
Tiggeloven, T. et al. Global-scale benefit–cost analysis of coastal flood adaptation to different flood risk drivers using structural measures. Nat. Hazards Earth Syst. Sci. 20 , 1025–1044 (2020).
Cao, W. et al. Increasing global urban exposure to flooding: an analysis of long-term annual dynamics. Sci. Total Environ. 817 , 153012 (2022).
Fang, J., Zhang, C., Fang, J., Liu, M. & Luan, Y. Increasing exposure to floods in China revealed by nighttime light data and flood susceptibility mapping. Environ. Res. Lett. 16 , 104044 (2021).
Shi, J., Cui, L. & Tian, Z. Spatial and temporal distribution and trend in flood and drought disasters in East China. Environ. Res. 185 , 109406 (2020).
Article CAS PubMed Google Scholar
Wang, Y. et al. Spatio-temporal changes of exposure and vulnerability to floods in China. Adv. Clim. Change Res. 5 , 197–205 (2014).
Du, S., He, C., Huang, Q. & Shi, P. How did the urban land in floodplains distribute and expand in China from 1992–2015? Environ. Res. Lett. 13 , 034018 (2018).
Dilling, L. et al. Is adaptation success a flawed concept? Nat. Clim. Change 9 , 572–574 (2019).
Rentschler, J. et al. Resilient Shores: Vietnam’s Coastal Development Between Opportunity and Disaster Risk (World Bank, 2020).
Lin, Y., McDermott, T. K. J. & Michaels, G. Cities and the Sea Level. CESifo Working Papers No. 8997. https://papers.ssrn.com/sol3/papers.cfm?abstract_id=3827610 (2021).
Hallegatte, S. How Economic Growth and Rational Decisions Can Make Disaster Losses Grow Faster Than Wealth. Policy Research Working Paper 5617. World Bank Group https://doi.org/10.1596/1813-9450-5617 (2011).
Sampson, C. C. et al. A high-resolution global flood hazard model. Water Resour. Res. 51 , 7358–7381 (2015).
Smith, A., Sampson, C. & Bates, P. Regional flood frequency analysis at the global scale. Water Resour. Res. 51 , 539–553 (2015).
Koks, E. E. et al. A global multi-hazard risk analysis of road and railway infrastructure assets. Nat. Commun. 10 , 2677 (2019).
Wing, O. E. J. et al. Validation of a 30 m resolution flood hazard model of the conterminous United States. Water Resour. Res. 53 , 7968–7986 (2017).
Bernhofen, M. V. et al. A first collective validation of global fluvial flood models for major floods in Nigeria and Mozambique. Environ. Res. Lett. 13 , 104007 (2018).
Yamazaki, D. et al. A high-accuracy map of global terrain elevations. Geophys. Res. Lett. 44 , 5844–5853 (2017).
Vousdoukas, M. I. et al. Global probabilistic projections of extreme sea levels show intensification of coastal flood hazard. Nat. Commun. 9 , 2360 (2018).
Vousdoukas, M. I. et al. Developments in large-scale coastal flood hazard mapping. Nat. Hazards Earth Syst. Sci. 16 , 1841–1853 (2016).
Tolman, H. L. User Manual and System Documentation of WAVEWATCH III version 3.14. Technical note. MMAB Contribution No. 276, 220 pp (2009).
Scussolini, et al. FLOPROS: an evolving global database of flood protection standards. Nat. Hazards Earth Syst. Sci. 16 , 1049–1061 (2016).
Blöschl, G. et al. Changing climate both increases and decreases European river floods. Nature 573 , 108–111 (2019).
Article ADS PubMed Google Scholar
Corbane, C., Florczyk, A., Pesaresi, M., Politis, P. & Syrris, V. GHS-BUILT R2018A - GHS built-up grid, derived from Landsat, multitemporal (1975-1990-2000-2014). European Commission, Joint Research Centre https://doi.org/10.2905/jrc-ghsl-10007 (2018).
Liu, X. et al. High-spatiotemporal-resolution mapping of global urban change from 1985 to 2015. Nat Sustain. 3 , 564–570 (2020).
Marconcini, M., Metz-Marconcini, A., Esch, T. & Gorelick, N. Understanding current trends in global urbanisation – the World Settlement Footprint suite. GI_Forum 9 , 33–38 (2021).
German Aerospace Center (DLR). EOC Geoservice https://geoservice.dlr.de/web/maps/eoc:wsfevolution (2021).
Download references
Acknowledgements
This study has benefited from feedback and input by L. Bernard, R. Damania, V. Deparday, K. Garrett, C. Gevaert, N. Holm-Nielsen, B. Jongman, N. Lozano Gracia, S. Ramesh, C. Riom and L. Southwood. It was supported by the Global Facility for Disaster Reduction and Recovery (GFDRR).
Author information
Authors and affiliations.
The World Bank, Washington, DC, USA
Jun Rentschler, Paolo Avner, Rui Su & Stéphane Hallegatte
German Aerospace Center (DLR), Munich, Germany
Mattia Marconcini
MindEarth, Biel, Switzerland
Mattia Marconcini & Emanuele Strano
Department of Marine Sciences, University of the Aegean, Mytilene, Greece
Michalis Vousdoukas
You can also search for this author in PubMed Google Scholar
Contributions
J.R. and P.A. led the conception, study design, analysis and drafting, with input from S.H., R.S. and M.M. M.M. and E.S. developed the WSF-Evo dataset, designed and implemented the computational process and performed sensitivity tests. M.V. developed the coastal flood-hazard data and performed sensitivity tests. All authors critically revised the manuscript and gave final approval for publication.
Corresponding author
Correspondence to Jun Rentschler .
Ethics declarations
Competing interests.
The authors declare no competing interests.
Peer review
Peer review information.
Nature thanks Thomas Corringham, Long Yang and the other, anonymous, reviewer(s) for their contribution to the peer review of this work. Peer reviewer reports are available.
Additional information
Publisher’s note Springer Nature remains neutral with regard to jurisdictional claims in published maps and institutional affiliations.
Extended data figures and tables
Extended data fig. 1 flood-exposed settlement extents as a share of overall settlements over time..
a , By region, settlement extent, normalized. b , By income group, settlement extent, normalized. Hazard classes are defined on the basis of the estimated inundation depth experienced during a 1-in-100-year flood: none (flood depths of 0 cm), low (up to 15 cm), moderate (between 15 and 50 cm), high (between 50 and 150 cm) and very high (over 150 cm).
Extended Data Fig. 2 Year-on-year settlement growth (%).
a , By region. b , By income groupings. Hazard classes are defined on the basis of the estimated inundation depth experienced during a 1-in-100-year flood: none (flood depths of 0 cm), low (up to 15 cm), moderate (between 15 and 50 cm), high (between 50 and 150 cm) and very high (over 150 cm).
Extended Data Fig. 3 Proportional representation of settlement extent.
a , Global settlement (1.3 million km 2 total in 2015). b , Settlement extent in high-hazard flood areas (144,600 km 2 total in 2015). c , New settlement extent in high-hazard flood areas (76,400 km 2 total during 1985–2015). These figures offer proportional representations of settlement areas in different countries. In each subfigure, the sum of all tiles corresponds to the total settlement extent (in km 2 ) specified in parentheses.
Extended Data Fig. 4 Sensitivity and robustness of estimates.
a , Flood-exposed settlement area for different inundation depth thresholds. b , IDC score. c , Vietnam: settlement expansion by hazard zones and flood return periods (1985–2015). China: settlement expansion by hazard zones and flood return periods (1985–2015).
Supplementary information
Supplementary information.
This Supplementary Information file contains the following three sections: relative accuracy of the WSF-Evo dataset (Kappa coefficients); settlement exposure growth at different flood return periods; country-level summary statistics. It includes two figures: Supplementary Fig. 1: Kappa coefficients for urban settlement datasets; Supplementary Fig. 2: settlement expansion by hazard zones and flood return periods (1985–2015) in Vietnam and China.
Reporting Summary
Supplementary data.
Subnational (admin-1) estimates.
Peer Review File
Rights and permissions.
Springer Nature or its licensor (e.g. a society or other partner) holds exclusive rights to this article under a publishing agreement with the author(s) or other rightsholder(s); author self-archiving of the accepted manuscript version of this article is solely governed by the terms of such publishing agreement and applicable law.
Reprints and permissions
About this article
Cite this article.
Rentschler, J., Avner, P., Marconcini, M. et al. Global evidence of rapid urban growth in flood zones since 1985. Nature 622 , 87–92 (2023). https://doi.org/10.1038/s41586-023-06468-9
Download citation
Received : 17 March 2022
Accepted : 21 July 2023
Published : 04 October 2023
Issue Date : 05 October 2023
DOI : https://doi.org/10.1038/s41586-023-06468-9
Share this article
Anyone you share the following link with will be able to read this content:
Sorry, a shareable link is not currently available for this article.
Provided by the Springer Nature SharedIt content-sharing initiative
This article is cited by
Rapid urban expansion and potential disaster risk on the qinghai-tibetan plateau in the 21st century.
- Chenglong Yin
- Annah Lake Zhu
Landscape Ecology (2024)
Floods have become less deadly: an analysis of global flood fatalities 1975–2022
- S. N. Jonkman
- L. M. Bouwer
Natural Hazards (2024)
Insurance retreat in residential properties from future sea level rise in Aotearoa New Zealand
- Belinda Storey
Climatic Change (2024)
By submitting a comment you agree to abide by our Terms and Community Guidelines . If you find something abusive or that does not comply with our terms or guidelines please flag it as inappropriate.
Quick links
- Explore articles by subject
- Guide to authors
- Editorial policies
Sign up for the Nature Briefing newsletter — what matters in science, free to your inbox daily.

Advertisement
Was Today’s Earthquake Connected to the Solar Eclipse?
The tidal forces on Earth grow as the sun, moon and Earth begin to align, a configuration that can lead to a solar eclipse. But the results of several studies of the relationship between earthquakes and tides are inconclusive, a geophysicist said.
- Share full article

By Katrina Miller
- April 5, 2024
With a total solar eclipse set to pass through the United States on Monday, it is easy to imagine a linkage between unusual events in the heavens and on Earth. But geoscientists were cautious about making such a connection.
Earthquakes happen along fault lines, or cracks between two blocks of rock on Earth’s crust. Tides stretch and squish the land on Earth just as they contribute to waves in the ocean, and those tidal forces grow as the sun, moon and Earth begin to align — a configuration that sometimes creates a solar eclipse.
One theory is that this may introduce additional stress along Earth’s fault lines.
“We do know that the relative position of the Earth and the moon and the sun does exert tidal forces,” said William Frank, a geophysicist at the Massachusetts Institute of Technology. “And we know that changes the stress that can be on a fault that can host an earthquake.”
But the results of several studies of the relationship between earthquakes and tides are inconclusive, according to Seth Stein, a geophysicist at Northwestern University. “If there’s any effect, it would be incredibly weak,” he said.
Earthquakes are driven most often by the motion between two tectonic plates making up Earth’s crust — either when two plates slide along each other in opposite directions, or when one slides under the other.
Both types of movements introduce strain at the junction, which often gets relieved by an earthquake.
But at the moment, it’s difficult to say that plate motion was responsible for the quake that shook the Northeast Friday morning.
“It’s not quite as obvious, because there is no tectonic plate boundary that is active,” Dr. Frank said.
Still, he added, fault lines from past activity are everywhere on Earth’s crust.
“Some of these faults can still be storing stress and be closure to failure,” he said. “And it can just require a little bit more to push it over the edge.”
Katrina Miller is a science reporting fellow for The Times. She recently earned her Ph.D. in particle physics from the University of Chicago. More about Katrina Miller
Our Coverage of the Total Solar Eclipse
Anticipation and Anxiety Build: Across parts of the United States, Mexico and Canada, would-be eclipse-gazers are on the move for what could be a once-in-a-lifetime event .
Awaiting a Moment of Awe: Millions of people making plans to be in the path of the solar eclipse know it will be awe-inspiring. What is that feeling ?
The Eclipse Chaser: A retired astrophysicist known as “Mr. Eclipse” joined “The Daily” to explain why these celestial phenomena are such a wonder to experience .
Historic Photos: From astronomers with custom-built photographic equipment to groups huddled together with special glasses, here’s what solar eclipse-gazing has looked like for the past two centuries .
Hearing the Eclipse: A device called LightSound is being distributed to help the blind and visually impaired experience what they can’t see .
Animal Reactions: Researchers will watch if animals at zoos, homes and farms act strangely when day quickly turns to night.
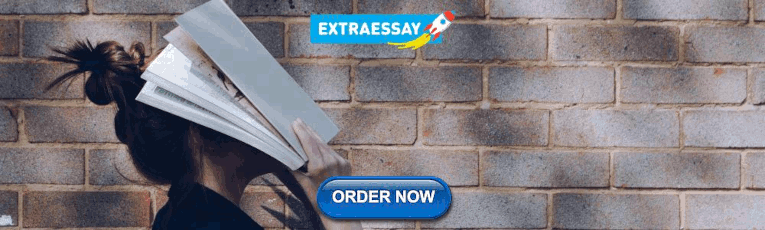
IMAGES
VIDEO
COMMENTS
Effects. In some areas, floodwater was between 3-4.5m deep. Floods in the southern Indian state of Kerala have killed more than 410 people since June 2018 in what local officials said was the worst flooding in 100 years. Many of those who died had been crushed under debris caused by landslides.
Building on an ethnographic case-study of one community in the County of Berkshire, England, we examine how two local flood groups reproduce local flood history and identify how these reproductions affect the sustainability of their flood management initiatives. ... while major floods are likely to foster formation of new social entities ...
One study projects that the global number of flood-exposed people will reach 1.3 billion by 2050 20, but our study shows that this threshold has already been exceeded by at least 39%.
The year through May 2019 was the wettest 12-month period on record in the United States, according to the National Oceanic and Atmospheric Administration. Nearly 38 inches of water fell, almost ...
Across case studies where two similar floods occurred in the same region, with the second flood causing substantially lower damage 15, the damage reduction is mainly attributed to substantial ...
We selected these cities as case study subjects for one or more of the following reasons: ... The United States and its cities have faced major floods and hurricanes - including Michael, Florence, Sandy, Katrina, Rita, Harvey, and Irma. The 1927 Flood of Mississippi led to enactment of the Flood Control Act in 1936, which recognized the need ...
Although a previous analysis of eight case studies 21 identified vulnerability as a key to reduction of flood impacts, ... One unprecedented pluvial flood is the 2014 event in the city of Malmö ...
In this case study we simulate floods at the most granular level (up to two-by-two-meter resolution) and explore how flood risk may evolve for Ho Chi Minh City (HCMC) and Bristol (See sidebar, "An overview of the case study analysis"). Our aim is to illustrate the changing extent of flooding, the landscape of human exposure, and the ...
By Danielle Torrent Tucker. In a new study, Stanford researchers report that intensifying precipitation contributed one-third of the financial costs of flooding in the United States over the past ...
Decades ago, a George Washington University study identified Tulsa as the most frequently flooded city in the United States. The city was built on the banks of the Arkansas River. It is located within "Tornado Alley," and it regularly experiences severe storms. The city flooded every few years in the 1960s and 1970s; in 1984, a major flood ...
Case study: Diagnosing China's prevailing urban flooding—Causes, challenges, and solutions. ... In order to safely convey major floods to the receiving streams or rivers without adverse impact to their regulatory floodplains, major flood conveyance and detention facilities would be needed. ... so one can hardly be surprised if unmanaged ...
Thus, it causes an abrupt increase in water volume that exceeds the river basin capacity. New development such as housing ranging from the hill to the valley is one of the major causes of increased flooding in the study area. What determines one's vulnerability is the gender roles, place, employment, health care, income and social status.
Major freshwater flood events from 2004 to 2014 cost an average of $9 billion in direct damage and 71 lives annually. These figures do not include the cumulative costs of frequent, small floods, which can be similar to those of infrequent extreme floods. ... These case study/information gathering sessions will provide information from federal ...
Heavy downpours across large parts of northern England led to surface water and river flooding in parts of Yorkshire, Nottinghamshire, Greater Manchester, Derbyshire and Lincolnshire. According to the Met Office, on Thursday 7th November 2019 over half of the average rainfall for the whole of November fell in parts of the Midlands and Yorkshire.
Flood risk and flood management: Introduction. Flooding 1: Causes of river floods. Flooding 2: Investigating the effects of river floods. Flooding 4: Managing the upper drainage basin. Flooding 5: Sustainable Drainage Systems (SuDS) Flooding 6: Managing river floods - exploring the role of the Environment Agency.
On 20 July 2021, an extreme rainstorm battered Zhengzhou in China's Henan Province, killing 302 people, including 14 individuals who drowned in a subway tunnel and 6 who drowned in a road tunnel. As the global climate warms, extreme weather events similar to the Zhengzhou flood will become more frequent, with increasingly catastrophic consequences for society. Taking a case study-based ...
This study integrates geocoded mortality records from 573 major flood disasters with population and economic data to perform generalized linear mixed regression modelling.
China: A Case Study of Flooding Severity and Landscape Characteristics Yanbo Duan 1 , Y u Gary Gao 2 , Y usen Zhang 1 , Huawei Li 1 , Zhonghui Li 3 , Ziying Zhou 1,4 , Guohang T ian 1, *
The study contends that major flooding can occur even in marginal flow conditions in the catchment when there is tidal influence. ... A major flood event occurred in December 2015 and was reported as one of the most disastrous floods in the history of the region. ... Gupta AK (2009) Urban floods: case study of Mumbai. Disaster Dev 3(2):99 ...
1 Introduction. Floods are one of the major sources of anthropological and ecological destruction. It affects the socio-economic conditions, worsens public health, generates unemployment, damages the ecosystem, etc. (Allaire, 2018; Parida, 2019).Currently, public and private institutions are struggling to formulate and evaluate risk management and adjustment strategies, involving systems for ...
determining flood cresting at Hurricane Katrina (2005), estimating flow rate and possible mitigation strategies at the Oso mudslides (2013), and the Louisiana floods (2016, unpublished). Murphy in [10] gives 3 case studies of emergency informatics for floods, one of which is the use of a SUAS for a missing persons search after a major flood of
The abandonment of inland areas has become a major demographical challenge, causing a condition of local fragility in terms of spatial marginalization. To deal with this issue in Italy, a number of policy actions have been implemented over the time, namely the National Strategy for Inland Areas, established a decade ago, and more recently the Next Generation EU (NGEU) to foster local economic ...
Kerr followed his father into medicine, and in the last 10 years he has hired a permanent research team that expanded studies on deathbed visions to include interviews with patients receiving ...
One study using coarse flood data projected that the global number of flood-exposed people will reach 1.3 billion by 2050 (ref. 28), but a more recent high-resolution study showed that this ...
The tidal forces on Earth grow as the sun, moon and Earth begin to align, a configuration that can lead to a solar eclipse. But the results of several studies of the relationship between ...