Thank you for visiting nature.com. You are using a browser version with limited support for CSS. To obtain the best experience, we recommend you use a more up to date browser (or turn off compatibility mode in Internet Explorer). In the meantime, to ensure continued support, we are displaying the site without styles and JavaScript.
- View all journals
- Explore content
- About the journal
- Publish with us
- Sign up for alerts
- Perspective
- Published: 23 December 2022
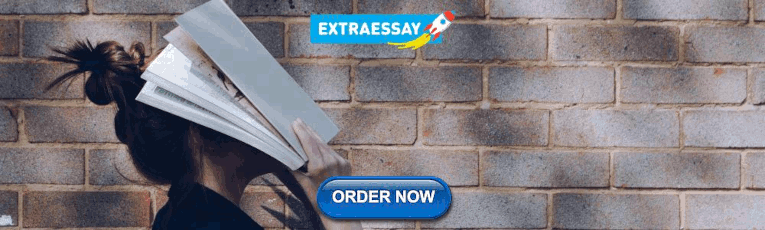
Advancing bio-based materials for sustainable solutions to food packaging
- Blaise L. Tardy 1 , 2 na1 ,
- Joseph J. Richardson 3 , 4 na1 ,
- Luiz G. Greca 1 na1 ,
- Junling Guo ORCID: orcid.org/0000-0002-2948-880X 5 , 6 ,
- Julien Bras 7 &
- Orlando J. Rojas ORCID: orcid.org/0000-0003-4036-4020 1 , 6
Nature Sustainability volume 6 , pages 360–367 ( 2023 ) Cite this article
3640 Accesses
26 Citations
8 Altmetric
Metrics details
- Engineering
- Research management
The unprecedented accumulation of plastic waste forms a serious threat to the biosphere, and current recycling efforts are not living up to their promise. Replacements for synthetic plastics are therefore critically needed, which has led to a rapid growth in research surrounding the development of sustainable materials, such as bioproducts. Still, commercialization has been limited, as knowledge gaps separating publicly funded research from industrial implementation need to be overcome. The food-packaging sector is currently undergoing drastic transformations in phasing out plastics and can therefore provide a blueprint for catalysing the adoption of bioproducts that could be applicable to other sectors.
This is a preview of subscription content, access via your institution
Access options
Access Nature and 54 other Nature Portfolio journals
Get Nature+, our best-value online-access subscription
24,99 € / 30 days
cancel any time
Subscribe to this journal
Receive 12 digital issues and online access to articles
111,21 € per year
only 9,27 € per issue
Buy this article
- Purchase on Springer Link
- Instant access to full article PDF
Prices may be subject to local taxes which are calculated during checkout
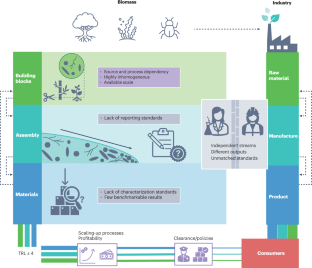
Similar content being viewed by others
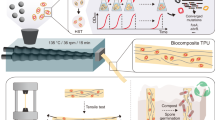
Biocomposite thermoplastic polyurethanes containing evolved bacterial spores as living fillers to facilitate polymer disintegration
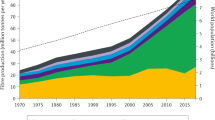
The environmental price of fast fashion
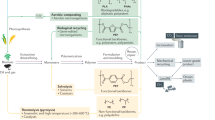
Bioplastics for a circular economy
Geyer, R., Jambeck, J. R. & Law, K. L. Production, use, and fate of all plastics ever made. Sci. Adv. 3 , e1700782 (2017).
Article Google Scholar
de Souza Machado, A. A., Kloas, W., Zarfl, C., Hempel, S. & Rillig, M. C. Microplastics as an emerging threat to terrestrial ecosystems. Glob. Change Biol. 24 , 1405–1416 (2018).
Cole, M., Lindeque, P., Halsband, C. & Galloway, T. S. Microplastics as contaminants in the marine environment: a review. Mar. Pollut. Bull. 62 , 2588–2597 (2011).
Article CAS Google Scholar
Zhang, J., Wang, L., Trasande, L. & Kannan, K. Occurrence of polyethylene terephthalate and polycarbonate microplastics in infant and adult feces. Environ. Sci. Technol. Lett. 8 , 989–994 (2021).
Li, T. et al. Developing fibrillated cellulose as a sustainable technological material. Nature 590 , 47–56 (2021).
Ates, B., Koytepe, S., Ulu, A., Gurses, C. & Thakur, V. K. Chemistry, structures, and advanced applications of nanocomposites from biorenewable resources. Chem. Rev. 120 , 9304–9362 (2020).
Virtanen, S., Chowreddy, R. R., Irmak, S., Honkapää, K. & Isom, L. Food industry co-streams: potential raw materials for biodegradable mulch film applications. J. Polym. Environ. 25 , 1110–1130 (2017).
Circular Economy Action Plan (European Commission, 2020); https://doi.org/10.2775/855540
Stahel, W. R. The circular economy. Nature 531 , 435–438 (2016).
Pauliuk, S. Making sustainability science a cumulative effort. Nat. Sustain. 3 , 2–4 (2020).
Moradali, M. F. & Rehm, B. H. A. Bacterial biopolymers: from pathogenesis to advanced materials. Nat. Rev. Microbiol. 18 , 195–210 (2020).
Kaur, L., Khajuria, R., Parihar, L. & Singh, G. D. Polyhydroxyalkanoates: biosynthesis to commercial production—a review. J. Microbiol. Biotechnol. Food Sci. 6 , 1098–1106 (2017).
Jabeen, N., Majid, I. & Nayik, G. A. Bioplastics and food packaging: a review. Cogent Food Agric. 1 , 1117749 (2015).
Yan, N. & Chen, X. Sustainability: don’t waste seafood waste. Nature 524 , 155–157 (2015).
Domard, A. A perspective on 30 years research on chitin and chitosan. Carbohydr. Polym. 84 , 696–703 (2011).
Tardy, B. L. et al. Deconstruction and reassembly of renewable polymers and biocolloids into next generation structured materials. Chem. Rev. 121 , 14088–14188 (2021).
Sachs, J. D. et al. Six transformations to achieve the Sustainable Development Goals. Nat. Sustain. 2 , 805–814 (2019).
Jones, M., Gandia, A., John, S. & Bismarck, A. Leather-like material biofabrication using fungi. Nat. Sustain. 4 , 9–16 (2020).
Zhao, X., Cornish, K. & Vodovotz, Y. Narrowing the gap for bioplastic use in food packaging: an update. Environ. Sci. Technol. 54 , 4712–4732 (2020).
Camberato, J. J., Gagnon, B., Angers, D. A., Chantigny, M. H. & Pan, W. L. Pulp and paper mill by-products as soil amendments and plant nutrient sources. Can. J. Soil Sci. 86 , 641–653 (2006).
Faria, M. et al. Minimum information reporting in bio–nano experimental literature. Nat. Nanotechnol. 13 , 777–785 (2018).
Schultz, D. & Campeau, L. C. Harder, better, faster. Nat. Chem. 12 , 661–664 (2020).
Debecker, D. P. et al. Shaping effective practices for incorporating sustainability assessment in manuscripts submitted to ACS Sustainable Chemistry & Engineering : catalysis and catalytic processes. ACS Sustain. Chem. Eng. 9 , 4936–4940 (2021).
Xia, Q. et al. A strong, biodegradable and recyclable lignocellulosic bioplastic. Nat. Sustain. 4 , 627–635 (2021).
Vollmer, I. et al. Beyond mechanical recycling: giving new life to plastic waste. Angew. Chem. Int. Ed. 59 , 15402–15423 (2020).
RameshKumar, S., Shaiju, P., O’Connor, K. E. & P, R. B. Bio-based and biodegradable polymers—state-of-the-art, challenges and emerging trends. Curr. Opin. Green Sustain. Chem. 21 , 75–81 (2020).
Villanueva, A. & Wenzel, H. Paper waste—recycling, incineration or landfilling? A review of existing life cycle assessments. Waste Manage. 27 , S29–S46 (2007).
Melo, F. P. L. et al. Adding forests to the water–energy–food nexus. Nat. Sustain. 4 , 85–92 (2021).
Wyser, Y. & Shires, D. Increasing the quality and impact of manuscripts in the field of new materials. Packag. Technol. Sci. 32 , 3–5 (2019).
Kinnunen, P. et al. Local food crop production can fulfil demand for less than one-third of the population. Nat. Food 1 , 229–237 (2020).
Verghese, K., Lewis, H., Lockrey, S. & Williams, H. Packaging’s role in minimizing food loss and waste across the supply chain. Packag. Technol. Sci. 28 , 603–620 (2015).
Wang, J. et al. Moisture and oxygen barrier properties of cellulose nanomaterial-based films. ACS Sustain. Chem. Eng. 6 , 49–70 (2018).
Tardy, B. L. et al. Exploiting supramolecular interactions from polymeric colloids for strong anisotropic adhesion between solid surfaces. Adv. Mater. 32 , 1906886 (2020).
Sev, A. How can the construction industry contribute to sustainable development? A conceptual framework. Sustain. Dev. 17 , 161–173 (2009).
Agarwal, J., Sahoo, S., Mohanty, S. & Nayak, S. K. Progress of novel techniques for lightweight automobile applications through innovative eco-friendly composite materials: a review. J. Thermoplast. Compos. Mater. 33 , 978–1013 (2020).
Jabbour, L., Bongiovanni, R., Chaussy, D., Gerbaldi, C. & Beneventi, D. Cellulose-based Li-ion batteries: a review. Cellulose 20 , 1523–1545 (2013).
Agate, S., Joyce, M., Lucia, L. & Pal, L. Cellulose and nanocellulose-based flexible-hybrid printed electronics and conductive composites—a review. Carbohydr. Polym. 198 , 249–260 (2018).
Blankenship, R. E. et al. Comparing photosynthetic and photovoltaic efficiencies and recognizing the potential for improvement. Science 332 , 805–809 (2011).
Ioannidis, J., Kim, B. & Trounson, A. How to design preclinical studies in nanomedicine and cell therapy to maximize the prospects of clinical translation. Nat. Biomed. Eng. 2 , 797–809 (2018).
Sumner, L. W. et al. Proposed minimum reporting standards for chemical analysis: Chemical Analysis Working Group (CAWG) Metabolomics Standards Initiative (MSI). Metabolomics 3 , 211–221 (2007).
Yam, K. The Wiley Encyclopedia of Packaging Technology (John Wiley & Sons, 2010).
Download references
Acknowledgements
This work received funding from the European Research Council under the European Union’s Horizon 2020 research and innovation programme (grant agreement no. 788489, ‘BioElCell’). J.J.R. is the recipient of an Australian Research Council Future Fellowship (project no. FT210100669) funded by the Australian government and JSPS Fellowship P20373 from the Japanese Society for the Promotion of Science. B.L.T. is the recipient of the Khalifa University of Science and Technology (KUST) Faculty Startup Project (Project: FSU-2022-021).
Author information
These authors contributed equally: Blaise L. Tardy, Joseph J. Richardson, Luiz G. Greca.
Authors and Affiliations
Department of Bioproducts and Biosystems, School of Chemical Engineering, Aalto University, Aalto, Finland
Blaise L. Tardy, Luiz G. Greca & Orlando J. Rojas
Department of Chemical Engineering, Khalifa University, Abu Dhabi, United Arab Emirates
Blaise L. Tardy
Department of Materials Engineering, School of Engineering, University of Tokyo, Tokyo, Japan
Joseph J. Richardson
School of Engineering, RMIT University, Melbourne, Victoria, Australia
BMI Center for Biomass Materials and Nanointerfaces, College of Biomass Science and Engineering, Sichuan University, Chengdu, China
Junling Guo
Bioproducts Institute, Department of Chemical and Biological Engineering, Department of Chemistry, and Department of Wood Science, University of British Columbia, Vancouver, British Columbia, Canada
Junling Guo & Orlando J. Rojas
Univ. Grenoble Alpes, CNRS, Grenoble INP (Institute of Engineering Univ. Grenoble Alpes), LGP2, Grenoble, France
Julien Bras
You can also search for this author in PubMed Google Scholar
Contributions
B.L.T., L.G.G., J.J.R. and J.B. contributed to the conceptualization, investigation and writing of the original draft. J.G. and O.J.R. discussed and edited the manuscript.
Corresponding author
Correspondence to Blaise L. Tardy .
Ethics declarations
Competing interests.
The authors declare no competing interests.
Peer review
Peer review information.
Nature Sustainability thanks Ramesh P. Babu, Run-Cang Sun and Yael Vodovotz for their contribution to the peer review of this work.
Additional information
Publisher’s note Springer Nature remains neutral with regard to jurisdictional claims in published maps and institutional affiliations.
Supplementary information
Supplementary information.
Supplementary Tables 1–3, Fig. 1 and Discussions 1–6.
Rights and permissions
Springer Nature or its licensor (e.g. a society or other partner) holds exclusive rights to this article under a publishing agreement with the author(s) or other rightsholder(s); author self-archiving of the accepted manuscript version of this article is solely governed by the terms of such publishing agreement and applicable law.
Reprints and permissions
About this article
Cite this article.
Tardy, B.L., Richardson, J.J., Greca, L.G. et al. Advancing bio-based materials for sustainable solutions to food packaging. Nat Sustain 6 , 360–367 (2023). https://doi.org/10.1038/s41893-022-01012-5
Download citation
Received : 01 June 2021
Accepted : 20 October 2022
Published : 23 December 2022
Issue Date : April 2023
DOI : https://doi.org/10.1038/s41893-022-01012-5
Share this article
Anyone you share the following link with will be able to read this content:
Sorry, a shareable link is not currently available for this article.
Provided by the Springer Nature SharedIt content-sharing initiative
Quick links
- Explore articles by subject
- Guide to authors
- Editorial policies
Sign up for the Nature Briefing newsletter — what matters in science, free to your inbox daily.

- Reference Manager
- Simple TEXT file
People also looked at
Review article, bio-based smart materials for food packaging and sensors – a review.

- 1 Microelectronics Research Unit, Faculty of Information Technology and Electrical Engineering, University of Oulu, Oulu, Finland
- 2 DiSTAS - Department for Sustainable Food Process, Università Cattolica del Sacro Cuore, Piacenza, Italy
- 3 Bio Base Europe Pilot Plant, Ghent, Belgium
Food industry must guarantee food safety and seek sustainable solutions for increasing shelf life and decreasing food waste. Bio-based smart packaging is a potential option, where sustainability and real-time monitoring of food quality are combined assuring health safety and providing economic and environmental benefits. In this context, bio-based refers not only to packaging materials that are from renewable sources and biodegradable, but also to the sensor elements. The scope of this review is to explore the state-of-the-art of bio-based polymers used as food contact materials and to highlight the potential of natural compounds for sensing chemical and physical changes of the environment to monitor the food quality. Finally, different sustainability aspects of the bio-based materials are discussed.
Introduction
Busy lifestyles and growing urban populations mean an increasing demand for food that is fresh, healthy, convenient, and fast. One of the key drivers of this growth is the world’s rising population which by the year 2050 will reach 9.7 billion people with increase of 26% ( The United Nations, 2019 ). With the global population rising, wastage of food including 47% of all fruit and vegetables and 12% of meat and animal products, is one of the greatest challenges to achieve food security ( Food and Agriculture Organization of the United Nations, 2019a ). Although food is our basic necessity, its production, processing, transportation and storage are rather complex from many aspects and need to fulfill a number of criteria to ensure the health and environmental safety and economic feasibility.
Foods pose potential danger of diseases due to bacterial ( Salmonella, Campylobacter, Listeria , and Cholera ), viral (Norovirus, Hepatitis A), parasite (tapeworms, trematodes, Ascaris, Cryptosporidium, Entamoeba histolytica , and Giardia ), fungal ( Aspergillus, Candida , and Fusarium ) and even prion infections as consequence of inappropriate handling and processing of the products causing foodborne diseases that affect ∼10% of global population with a death toll of 420,000 deaths each year. Chemical contaminants, which may even accumulate in various food chains, represent further risks. These include phytochemical residues, mycotoxins, marine toxins from algae, cyanogenic glycosides from plants, and different metabolites from products aging and decaying (ethanol, putrescine, cadaverine, histamine, ethylene etc.) but also environmental and industrial pollutants, e.g., dioxins, polychlorinated biphenyls and heavy metals (Pb, Cd, and Hg) ( World Health Organization, 2019 ). Another issue in the context of food safety is deliberate fraud to counterfeit the origin, content or quality (i.e., expiration dates) of products ( Europol, 2015 ). Just diluting a high-quality wine with cheaper one mainly hurts the wallet and pride but more severe cases may endanger health permanently or cost lives ( Branigan, 2008 ). Furthermore, the flip side of food safety is food waste as 1/3 of all produced food is lost or goes into waste ( Food and Agriculture Organization of the United Nations, 2019a ) meaning safe and edible food products are thrown away although the “best if used before” dates are only recommendations without information of the true status of the food. This is ethically and practically controversial as still today 820 billion people suffer from undernutrition and agricultural production would need to increase with 50% to feed the growing population by 2050 ( Food and Agriculture Organization of the United Nations, 2019b ).
As a partial solution to complex problems of food safety and decreasing unnecessary food waste is the selection and development of proper food packaging. The function of the food packages has been the same throughout history: to maintain hygiene, protect the food during transportations and storing, and ultimately to increase shelf life. However, modern technology and materials science have introduced new “smart” functions to food packages, which include advanced packaging materials with improved properties, and sensors that can monitor food quality ( Yam et al., 2005 ; Kuswandi et al., 2011 ). Over the years, several approaches toward smart packaging have been demonstrated including time-temperature indicators, modified atmosphere packaging sensors for CO 2 and O 2 monitoring, total volatile base nitrogen sensors to detect food decay, fruit ripeness indicators, pathogen sensors, and solutions for food tracking and authentication (RFID tags) ( Fuertes et al., 2016 ; Ghaani et al., 2016 ; Ahmed et al., 2018 ; Badia-Melis et al., 2018 ; Galstyan et al., 2018 ; Mustafa and Andreescu, 2018 ; Yousefi et al., 2019 ).
As of today, plastics (rigid and flexible) have the largest shares of the market in food packaging (37% market), followed by paper and board (34%), glass (11%), and metal (9%) ( Muncke, 2012 ). If we consider the properties of the given food packaging materials, the high market share of paper and board can be explained by the renewable source and recyclability, it is printable, wet and dry food can be stored in paper and board after laminating/covering process and in general paper and board are very suitable for mass production lowering the costs ( Kirwan, 2011 ). Containers made of glass, on the other hand, are among the oldest materials man has used as it can be shaped to practically any form, has high chemical resistance, is impermeable to gasses, absorbs UV and even parts of visible spectrum (amber glass, green and glass partially), is hygienic and reusable, and the consumers associate it to high-quality products ( Grayhurst and Girling, 2011 ). Metal is also an important food packaging material as it is durable withstanding packaging conditions in vacuum or under pressure and high temperature stabilization process for long shelf-life foods. Metal is reusable, UV-resistant and the food contact surface may be coated with different coatings in case the interaction between the product and the plain container would downgrade the shelf-life/quality of the product to an unacceptable level due to, e.g., metal surface corrosion or undesired food coloring as a consequence of combination of metal ions with the food components ( Oldring and Nehring, 2007 ). Typically metal containers or cans are made of steel (tin-coated or tin-free) or aluminum ( Kraus and Tarulis, 2009 ; Reingardt and Nieder, 2009 ; Robertson, 2012 ).
The most frequently used plastics in food packages in Europe are PP (19.3%), LDPE and LLDPE (17.5%), and PET (7.4%); and in fact, 39.9% of all produced plastics (61.8 million tons in Europe in 2018) goes to packaging in general ( PlasticsEurope, 2019a ). The success of plastic as packaging material can be explained by low cost, ease of modification from flexible films to rigid containers, strength, stability, light-weight, impermeability with gasses and many solvents, and enabled sterilization without affecting the food quality ( PlasticsEurope, 2019b ). Despite of numerous benefits, plastics are also problematic: annual global production of plastics is around 350 million tons of which only 1% is bio-based ( European Bioplastics e.V., 2020 ) and the rest is fossil-derived with large carbon footprint [6% of all produced oil goes to plastics, having a carbon footprint equivalent to the aviation sector ( World Economic Forum et al., 2016 )]. In addition, plastic pollution is alarming, as polymers do not degrade but break down to smaller pieces ending up in the air, soil and water as microplastics, found even in deep-sea amphipods ( Jamieson et al., 2019 ).
As a response to challenges associated with food safety, storage and transportation, there is a huge market need for more sustainable bio-based plastics and sensors that could alleviate the environmental, public health and economic burden caused by traditional materials. The scope of this short review is to collect the contemporary literature on bio-based smart food packages covering not only bio-based food packaging materials but also bio-based sensors for monitoring various physical, chemical and biological conditions of foodstuff.
Bio-Based Plastic Packaging Materials
Production data for bio-based and biodegradable plastics are available to a limited extent only, although production capacity data are more readily accessible. Currently the production capacities of bio-based and biodegradable plastics are low ( Figure 1 ), however, the market of some bio-based and/or biodegradable plastics are expected to grow significantly during the coming years (Bio-PET, PBS, and PLA) others are expected to consolidate (CA and Bio-PA) ( van den Oever et al., 2017 ). Overall, it is expected that the global bioplastics production capacity is set to increase from around 2.11 million tons in 2019 to approximately 2.43 million tons in 2024 ( Figure 2 ).
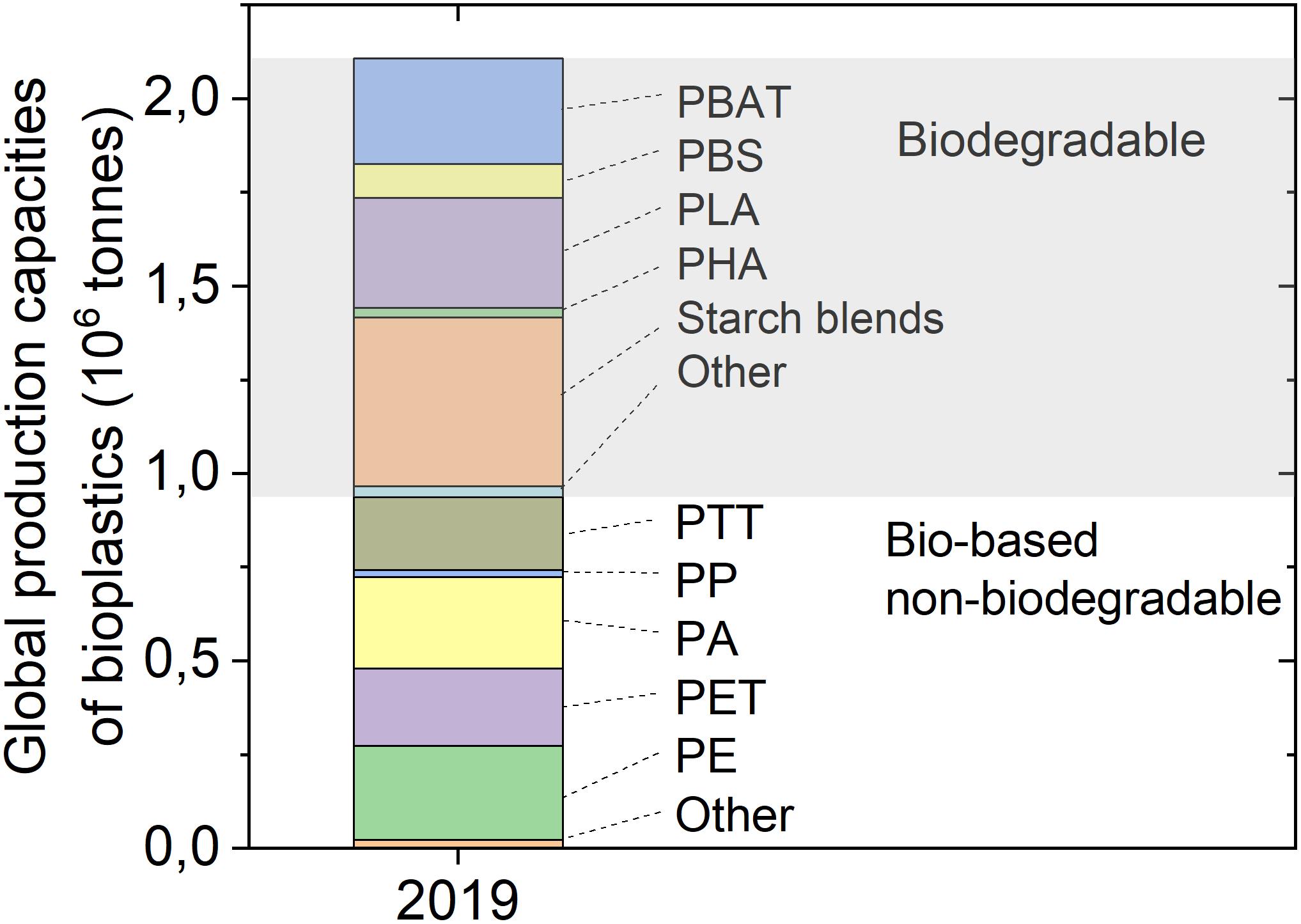
Figure 1. Current global production capacities of bioplastics by material type ( European Bioplastics e.V., 2020 ).
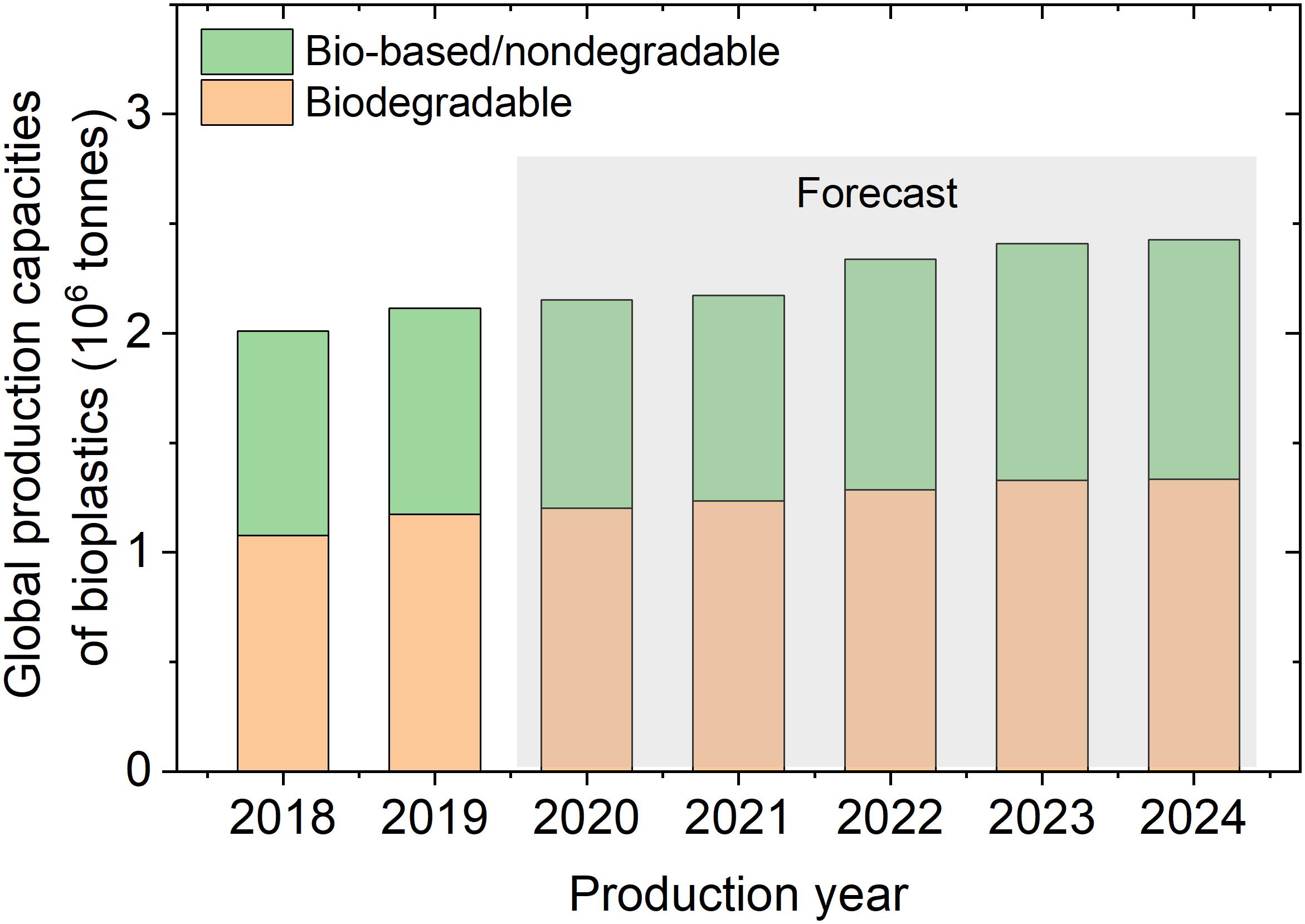
Figure 2. Current and forecast global production capacities of bioplastics ( European Bioplastics e.V., 2020 ).
A number of different routes have been developed during the past decade to produce bio-based materials [i.e., either made of bio-based source or it is biodegradable or contains both of these features ( European Bioplastics e.V., 2019 )] with a large variety of properties and applications areas ( Table 1 ). Among these, only a few families are made of renewable biomass and are biodegradable [e.g., cellulose and starch thermoplastics, PHAs, PLA, polyester amides ( Avérous, 2008 )], viz. bio-based polyethylene, PP, polyamide and polyethylene terephthalate are non-biodegradable, and PCLs and PVAs are from non-renewable resources ( Chen and Patel, 2012 ; Geueke, 2014 ; van Crevel, 2016 ). The main production routes are as follows:
1. Direct extraction of biopolymers such as starch and cellulose with subsequent thermopressing/molding to make thermoplastic starch polymers (TSPs) or using additional functionalization, e.g., acetylation, carboxymethylation and phosphorylation to produce CA, carboxymethyl cellulose and cellulose diphenyl-phosphate, respectively, which are then polymerized further ( Šešlija et al., 2018 ) or used as additives in polymers ( Weinmann and Cotton, 1958 ).
2. Hydrolysis to sugars followed by bacterial synthesis of polyesters, e.g., PHAs including PHB.
3. Conversion into sugars that are fermented to lactic acid followed by its direct polycondensation or by ring-opening condensation of lactide to PLA ( Avérous, 2008 ).
4. Chemical conversion into monomers followed by polymerization, e.g., amino acids obtained by hydrolysis and separation are polymerized with esters of lactonized unsaturated fatty acids in PEA synthesis.
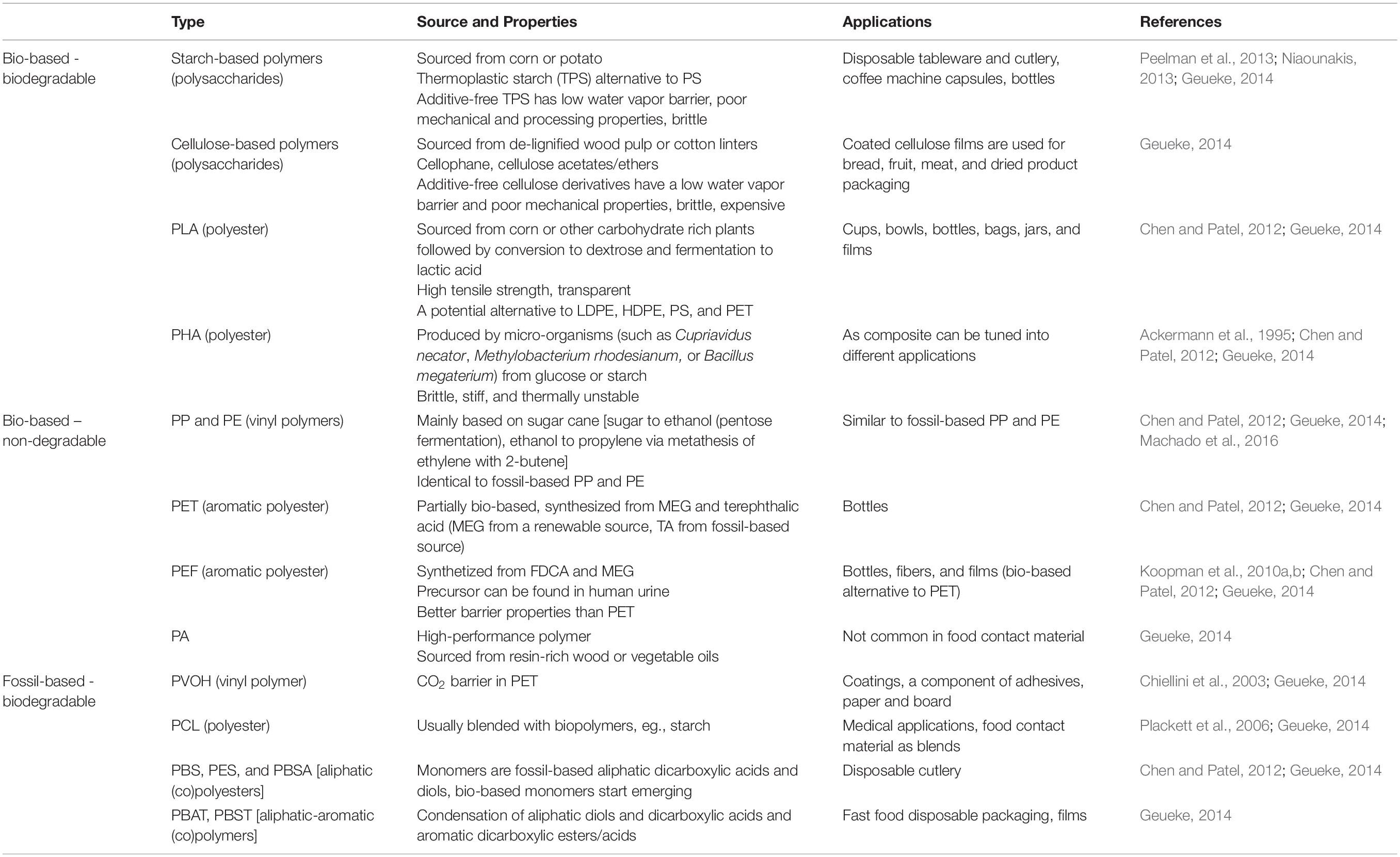
Table 1. Bio-based plastics in food packaging.
Based on the technical report published by Wageningen Food & Biobased Research in 2017 ( van den Oever et al., 2017 ), it has been shown that the bio-based and biodegradable plastics are currently more expensive than fossil-based plastics on weight basis ( Tables 2 , 3 ). However, specific material properties can allow costs reductions in the use or end-of-life phase. Further, the price of fossil-based plastics is dependent on oil prices and fluctuating with it, while in general the price of bio-based plastics depends on biomass prices that are more stable. When the production scale, conversion into final products and logistics become more favorable, it is expected that the prices of bio-based plastics will come down.
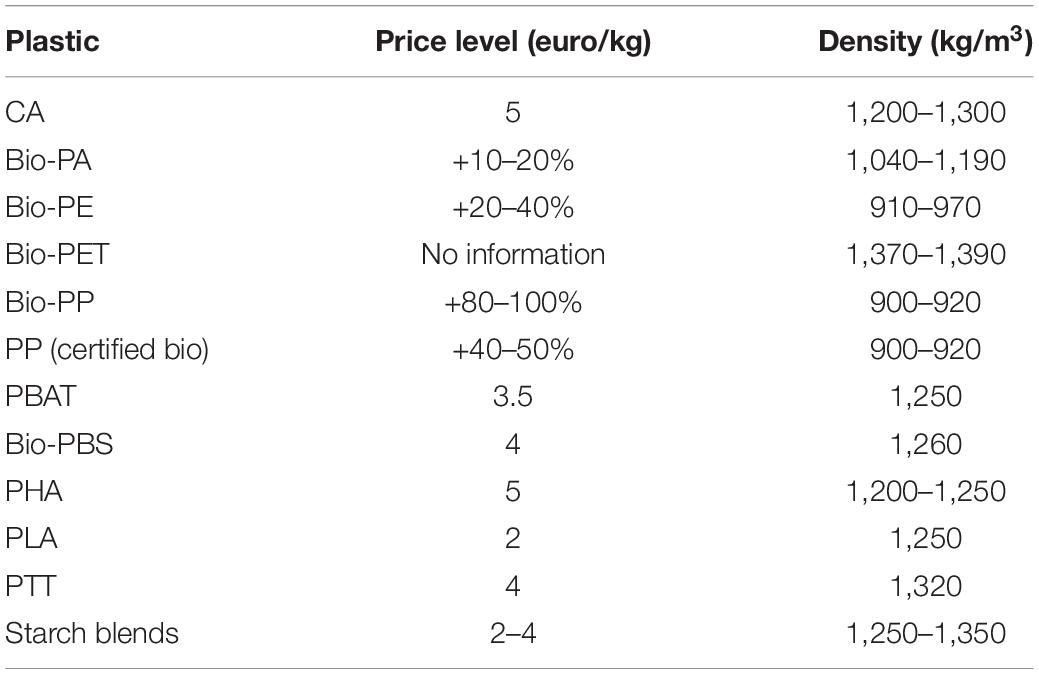
Table 2. Price level for bio-based and/or biodegradable plastics.
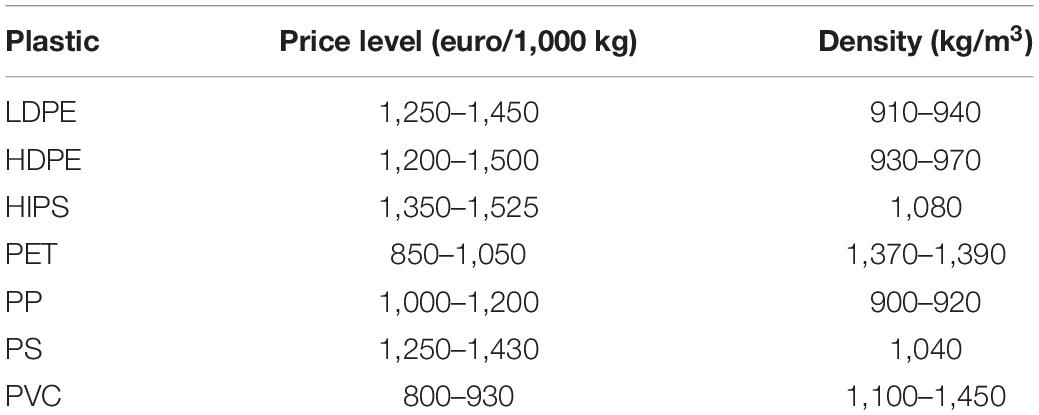
Table 3. Price level for fossil-based plastics.
In addition, since most bio-based plastics have a higher density, this directly contributes to their higher price. But there are exceptions when prices are compared on a product level. By selecting specific material properties and redesigning can allow material savings. For example, a traditional HIPS-based cup of 0.89 mm wall thickness could be down-gauged using impact modified PLA to 0.66 mm thickness ( Schut, 2016 ).
Bio-Based Smart Food Packages
Many new concepts in food packaging, like the smart functionalities, have been introduced during the last years in response to the increasing demand of ready-to-eat and higher quality foods ( Vanderroost et al., 2014 ).
Smart functionalities of food packages refer to active coatings and physical/chemical sensors combined with the packaging materials. The purpose of smart antimicrobial coatings is to mitigate the proliferation of various microbes thus prolonging the shelf-life of products, whereas sensors play role in monitoring physical and chemical conditions that influence or reflect the quality of the food products. These add-ons have inevitable positive health, environmental and socio-economic effects, which may be amplified even further by accomplishing the smart functions using renewable natural materials and robust technologies ( Arroyo et al., 2019 ).
Antimicrobial Films
As mentioned, active food packaging involves the use of polymeric films that act as a support for various active compounds such as natural extracts that can be incorporated during the manufacturing process of the packaging itself ( Kuorwel et al., 2015 ; Bassani et al., 2019 ). Antimicrobial incorporation may result in a material with antibacterial activity which can suppress the growth of bacteria on the material surface (according to the international norm ISO 22196:2011 – Measurement of antibacterial activity on plastics and other non-porous surfaces). In the food sector, a greater interest is toward materials enriched with antimicrobials so that the direct use of food additives in products is limited.
Antimicrobial materials act in two different ways. Antimicrobials can be incorporated into the film or coated either on the surface of the film or on the surface of the food (in the form of edible film). In both cases, the substance may migrate partially or completely through gradual diffusion into the food or headspace (which is typical for essential oils, for example) where it exerts its protective action, or it may not migrate, acting only when the food is in contact with the surface of the film and the target microorganism comes into direct contact with the film ( Vermeiren et al., 2002 ; Brockgreitens and Abbas, 2016 ) ( Figure 3 ).
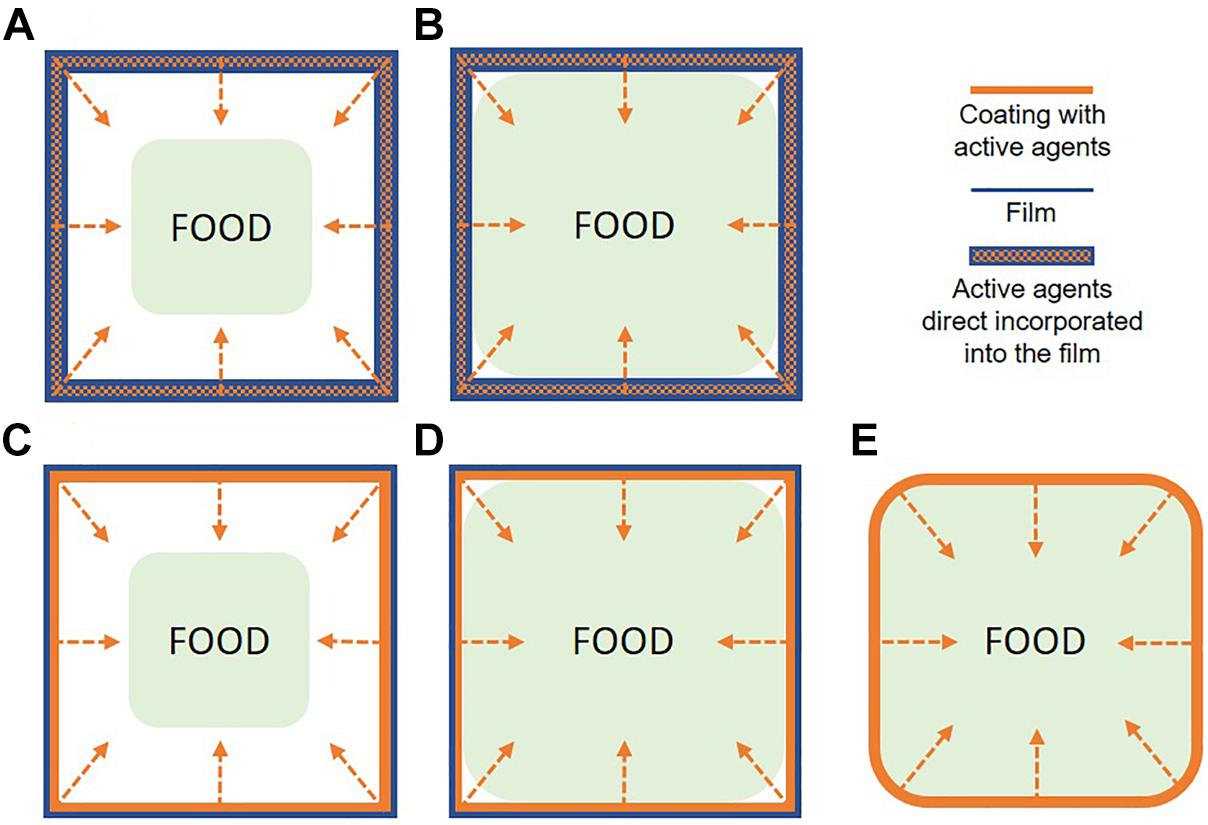
Figure 3. Different ways of incorporation and release of antimicrobial agents into food: (A) direct incorporation into the film before extrusion and migration via gradual diffusion from the material into the headspace; (B) direct incorporation into the film before extrusion and migration via gradual diffusion from the material into the food through direct contact; (C) surface coating on the film and migration via gradual diffusion from the material into the headspace; (D) surface coating on the film and migration via gradual diffusion from the material into the food through direct contact; (E) edible film and migration via gradual diffusion from the material into the food through direct contact.
In both the cases, this kind of packaging is called active packaging [Regulation (EC) No 450/2009 — active and intelligent materials and articles intended to come into contact with food].
Antimicrobial agents used for the preservation of foods are either chemically synthesized or extracted from biomass of plants, animals and microorganisms. Conventional chemical preservatives, including ethanol and other alcohols, organic acids, and their salts (benzoates, propionates, and sorbates) are the predominant food preservatives thanks to their low price and facility to use. However, research has been focusing on replacing them with natural antimicrobial agents such as enzymes, bacteriocins, chitin and its derivative chitosan extracted from crustacean shells, natural extracts, and essential oils ( Holley and Patel, 2005 ; Aider, 2010 ; Lei et al., 2014 ; van den Broek et al., 2015 ; Mlalila et al., 2018 ). Indeed, natural extracts (e.g., plant extracts or essential oils from different spices, plants, and fruits) have been recognized as potential antioxidant and antimicrobial agents. Some of the most successful examples of the incorporation of natural substances into films have involved grapefruit seed and green tea extracts, which have shown to be active as antioxidants and against different pathogens (e.g., Escherichia coli and Listeria spp.) ( Wang and Rhim, 2016 ; Wrona et al., 2017 ). Cinnamaldehyde, derived from cinnamon, was also studied for its bioactivity against E. coli and Salmonella spp. ( Ma Y. et al., 2018 ). Moreover, cinnamon oil in the PVA matrix showed repellent effect toward Plodia interpunctella larvae ( Jo et al., 2015 ) and in PP film inhibited the formation of molds ( Manso et al., 2015 ). However, clove and cinnamon in cassava starch films failed to show clear antimicrobial effect even though they reduced the water vapor transmission ( Kechichian et al., 2010 ). Another example was provided by Seydim and Sarikus (2006) who tested edible films made of whey protein isolate loaded with rosemary, oregano and garlic essential oils against E. coli, Staphylococcus aureus, Salmonella enteritidis, Listeria monocytogenes , and Lactobacillus plantarum . Oregano proved to be the most effective against bacteria, while rosemary showed no effect.
The most popular technique to include natural extracts into the final film formulation is the extrusion ( Gómez-Estaca et al., 2014 ). This technique involves the incorporation of the bioactive compounds before extrusion so that the high temperatures of extrusion (the exact values depend on the melting temperature of the processed polymer) allow their effective and homogeneous distribution in the film. However, this technique can often result in thermal degradation of the bioactive compounds and decrease in their activity. For instance, Ha et al. (2001) used high-temperature profile 160–190°C to extrude an antimicrobial LLDPE-based film resulting in high loss of grapefruit seed extract functionality up to complete loss of antimicrobial activity. For this reason, heat-sensitive bioactive agents (i.e., natural extracts) are preferably produced by non-heating method (e.g., electrospinning and surface coating). Among these methods, surface coating is a simple process based on low temperatures. However, this technique may suffer from poor adhesion to plastics and, if applied to make an active packaging, needs to be designed to be in direct contact with the food. Examples of antimicrobial-coated films include chitosan/essential oil-coated PP film ( Torlak and Nizamlioğlu, 2011 ), cinnamaldehyde, garlic oil and rosemary oil-coated PP/LDPE film ( Gamage et al., 2009 ), oregano essential oil and citral-coated PP/EVOH film ( Muriel-Galet et al., 2013 ), chitosan-coated plastic film ( Ye et al., 2008a , b ), and thyme and oregano-coated LDPE. Interestingly, as reported by Valderrama Solano and de Rojas Gante (2012) , antimicrobial films produced by elevated temperature processes showed better microbial inhibition compared to the ones obtained by the coating method. In particular, they found that antimicrobial films produced by extrusion method are more effective against E. coli, Salmonella typhimurium , and L. monocytogenes compared to ionizing-coated antimicrobial films with the identical amount of agent incorporated antimicrobial. The results suggest that the extrusion method allows a better incorporation of the active compounds on the polymer. Given the number of pros and cons highlighted by the literature for both the techniques, more studies comparing the efficacy of two methodologies will be needed in order to address future researches in this field. Indeed, there isn’t any large scale industrial production of active bio-based films yet. For this reason, an estimation of the cost of active films can be based on an average cost of commercial natural extract (about 100 €/kg even though it can greatly vary with extract type) and of PLA film (2 €/kg, see Table 2 ). Following the steps of the extrusion process, tested by Bassani et al. (2019) , with the inclusion of natural extracts encapsulated with β-cyclodextrins (370 €/kg), an estimation of the final price of active films was done resulting in about 6.4 €/kg. It is useful to point out that this evaluation was made considering an addition of encapsulated extract equal to 2% wt as maximum ( Bassani et al., 2019 ) and that this estimation already includes the costs necessary to encapsulate the extract by spray-drying technique.
Bio-Based Sensors
Bio-based sensors have at least one component from bio-based source which may either be the substrate (i.e., the plastics listed in the previous section) or the sensing element. Most of the sensors related to bio-based materials in food packaging are based on colorimetric detection of analytes.
Many fruits, berries, vegetables and flowers with colors covering practically the entire visible spectrum are dyed by natural compounds such as anthocyanins and curcumin known as natural pH indicators ( Yoshida et al., 2009 ; Silva-Pereira et al., 2015 ; Choi et al., 2017 ; Dudnyk et al., 2018 ; Majdinasab et al., 2018 ; Saliu and Pergola, 2018 ; Zhai et al., 2018 ; Kurek et al., 2019 ). Upon protonation/deprotonation of these molecules, their delocalized electronic structure rearranges and the change of the total number of resonant electrons as well as their confinement result in a change of their color ( Figure 4 ). For instance, Choi et al. (2017) demonstrated a pH sensor made of agar and potato starch with anthocyanin extracts from purple sweet potato that showed color variations at pH 2.0–10.0. Zhai et al. (2018) used a gelatin-gellan gum matrix with red radish anthocyanin having a slightly broader pH range from 2.0 to 12.0. In Table 4 more examples of bio-based sensors developed for food quality monitoring in recent years are listed.
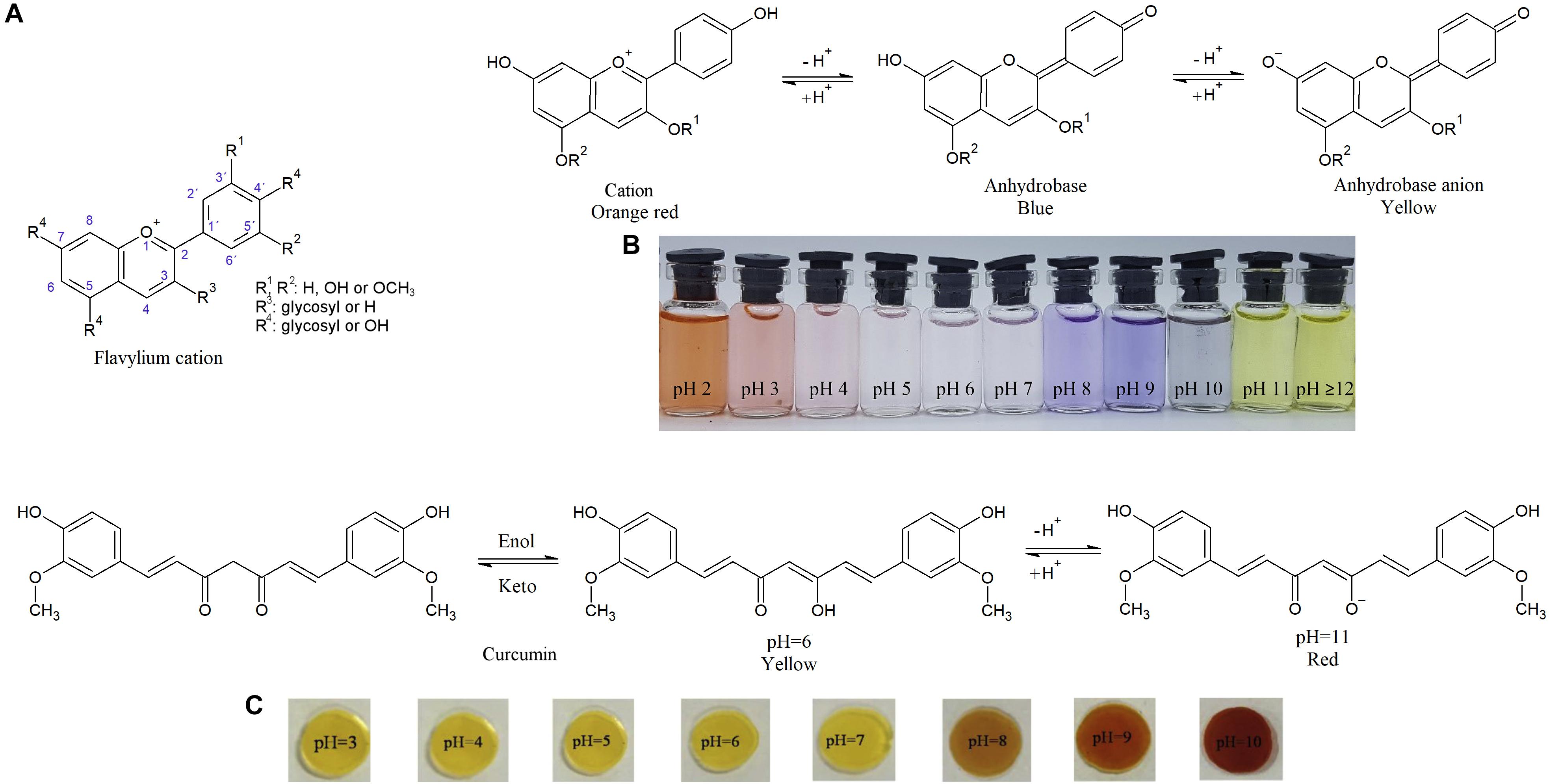
Figure 4. (A) Flavylium cation. In naturally occurring anthocyanidins, the terminating chemical groups (–H, –OH, and –OCH 3 ) in the 3, 5, 6, 7 and the 3′, 4′, and 5′ positions determine the original color of the molecule. pH-sensitivity and color change of (B) pelargonidin and (C) curcumin. [ (A) Reprinted from Phytochemistry 64(5), Kong et al. (2003) . Copyright (2020) with permission from Elsevier. (B) Reprinted with permission from Zhai et al. (2018) . Copyright (2020) American Chemical society. Reprinted from Spectrochim. Acta A 226, Chayavanich et al. (2020) Copyright (2020), with permission from Elsevier. (C) Reprinted from Food Hydrocolloid . 83, Liu et al. (2018) Copyright (2020), with permission from Elsevier].
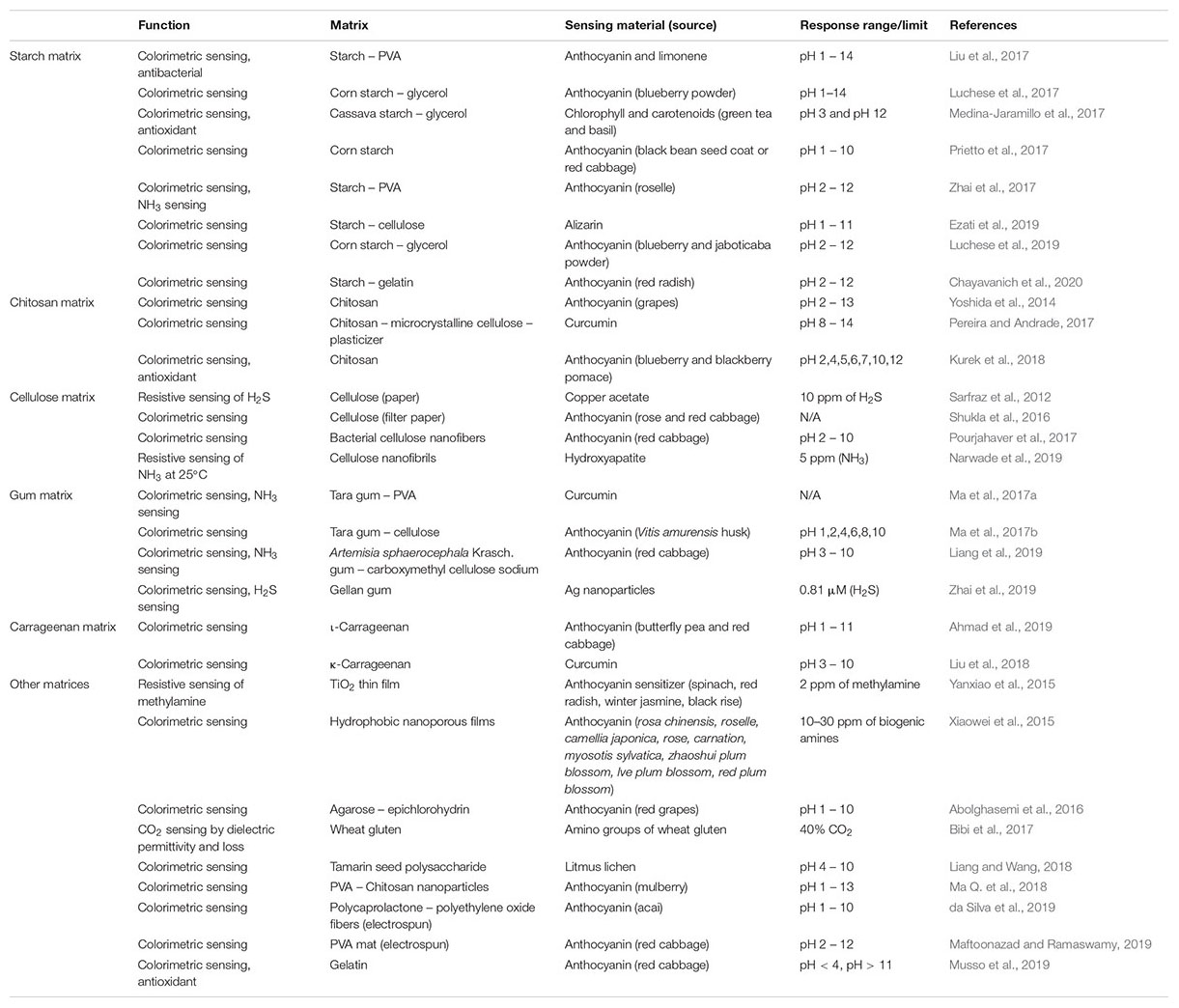
Table 4. Bio-based sensors developed for food monitoring.
Although colorimetric pH-sensitive sensors are typically not convenient for selective analysis, it is often sufficient to evaluate the food quality based on the change of the pH, as deteriorating proteins produce alkaline volatile nitrogen compounds (cadaverine, putrescine, histamine, and ammonia) ( Bulushi et al., 2009 ; Prester, 2011 ). Exploiting this indirect sensing mechanism, curcumin based indicator films, e.g., in gelatin ( Musso et al., 2017 ) and bacterial cellulose membranes ( Kuswandi et al., 2012 ), blueberry and red grape skin pomace in chitosan and carboxymethyl cellulose matrix ( Kurek et al., 2019 ), chitosan-corn starch film with red cabbage extract ( Silva-Pereira et al., 2015 ), alginate beads with red cabbage extract ( Majdinasab et al., 2018 ) as well as red cabbage extract in pectin films ( Dudnyk et al., 2018 ) have been shown as feasible indicators of meat, shrimp and fish spoilage by detecting amines and cyclic N-containing compounds. In a similar way, acidic CO 2 evolves during the metabolism of pathogens in the food thus lowering pH, which may be detected, e.g., by anthocyanin/polylysine in cellulose matrix in a reversible manner as demonstrated by Saliu and Pergola (2018) .
Other natural dyes such as chlorophyll and β-carotene might be also relevant for sensing since both structures are highly sensitive to oxidative species. Silva et al. (2017) showed that replacing the coordinated Mg 2+ with Zn 2+ in chlorophyll A, the fluorescence of the complex is faded when increasing the concentration of dissolved oxygen in the medium. The mechanism of luminescence suppression is suggested to be caused by an energy transfer to oxygen molecules that collide with the excited molecule. In the case of carotene, one may exploit several mechanisms for sensing. β-carotene is prone to oxidation and subsequent decomposition to shorter cleavage products leading to a gradual disappearance of the orange color ( Pénicaud et al., 2011 ).
Synthetic dyes based on various azo-compounds and polydiacetylenes also hold promise for chemical sensing in food packages. Azo-anthraquinone based dyes immobilized on paper (cellulose) as pH sensors working either in acidic or alkaline conditions, depending on the selected pigment, were shown by Zhang et al. (2019) . Selective amine sensing colorimetric indicators utilizing trifluoroacetyl azobenzene dyes developed by Mohr (2004a ; 2004b ), Reinert and Mohr (2008) ; Kirchner et al. (2006) have been exploited in colorimetric and electrochemical detection of ammonia, ethylamine, cadaverine and putrescine ( Lin et al., 2015 ). The carbonyl carbon of the trifluoroacetyl group is highly electron-deficient thus readily reacts with electron donors such as amines or alcohols. In the presence of amines (primary, secondary, or tertiary) it forms a hemiaminal group, i.e., the number of delocalized electrons in the diazobenzene backbone is decreased (so as the confinement length of electrons) resulting in a blue shift of optical absorption. Sensors printed on paper could detect vapors of the analytes having a concentration of 1.0–0.1 vol.%. Furthermore, highly sensitive ammonia sensors that operate even at very low temperatures (down to −20°C) were demonstrated by using polydiacetylenes that were polymerized in self-assembled vesicles stabilized with cellulose nanocrystals in the chitosan matrix. The sensing mechanism is based on a conformal change of the polydiacetylene backbone (from planar to non-planar) upon external stimuli such as pH, mechanical stress or temperature. Films of the sensors could detect 100 ppm ammonia ( Nquyen et al., 2019 ) ( Figure 5 ).
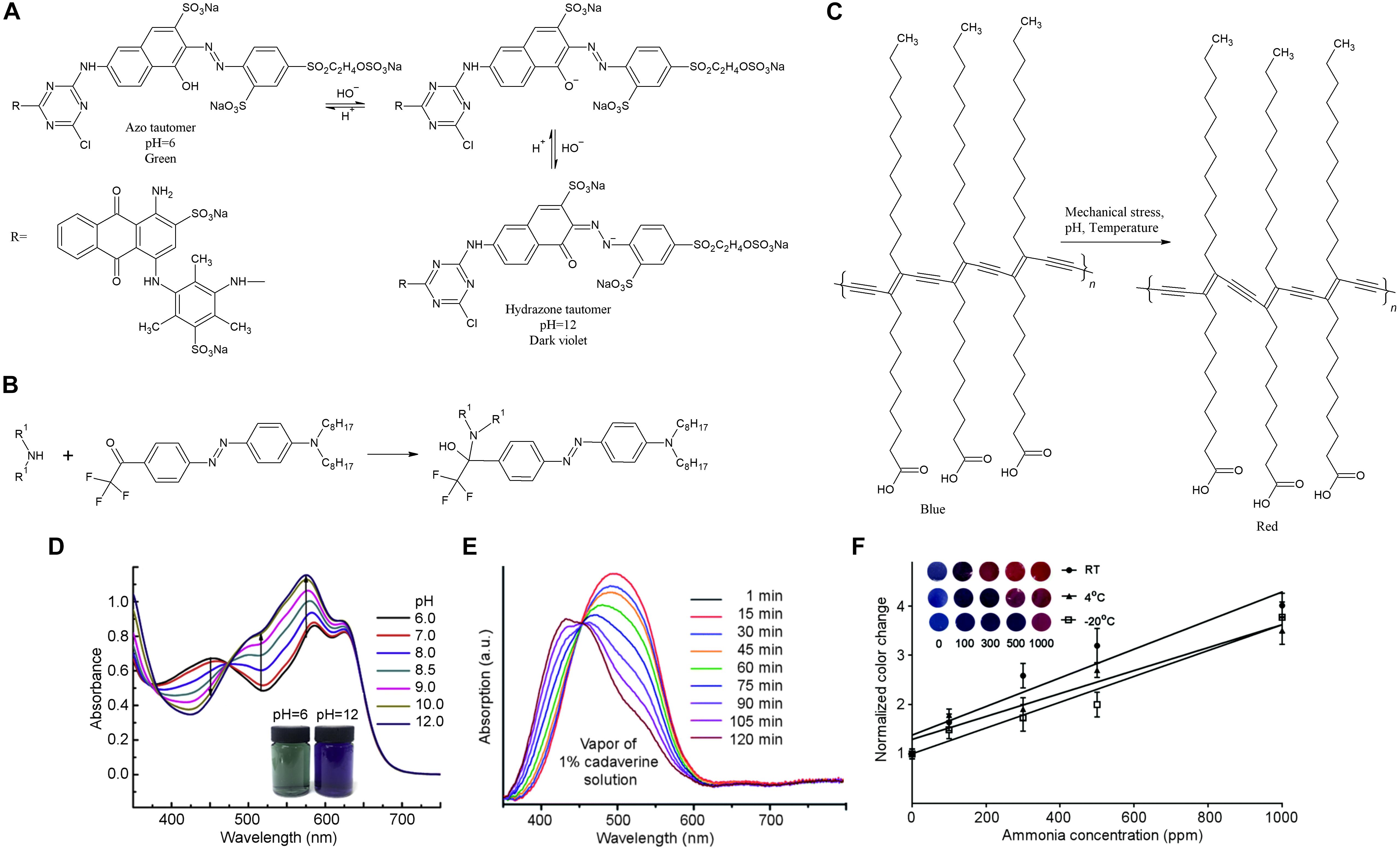
Figure 5. Reaction mechanisms of (A) anthraquinone and (B) trifluoroacetyl azo dyes and (C) polymerized self-assembled polydiacetylene vesicles and the corresponding color change during analyte sensing as displayed in panels (D–F) , respectively. Note, that the normalized color change in panel (F) is the ratio of red and blue components in the RGB coordinates in reference to the original values. [ (A,D) Reprinted from Sens. Actuat. B 286, Zhang et al. (2019) Copyright (2020), with permission from Elsevier. (B,E) Reproduced from Lin et al. (2015) . (C,F) Reproduced from Nquyen et al. (2019) with permission from The Royal Society of Chemistry.].
Colorimetric sensors/indicators may be also accomplished by using enzymatic processes, in which the color change is typically a function of temperature and time. Capitalizing on these, Yan et al. (2008) developed a temperature indicator that combines the coloration of iodine-starch clathrates and the influence of temperature on the hydrolysis of starch in the presence of amylase enzyme. From the kinetic reaction rates of hydrolysis (and the corresponding coloration of the clathrates), one may assess the time-temperature history of cooled products. Another example of time-temperature indicator based on enzymatic oxidation of ABTS [2,20-azino bis-(3-ethyl benzthiazoline-6-sulphonic acid] substrate resulting in green color was demonstrated by Rani and Abraham (2006) . By applying a fuse-type melting medium between peroxide and the mixture of the enzyme and substrate, the reaction starts only when the medium is warm enough to melt the separator thus enabling the mixing of the reactants. A similar melting fuse type of color indicator was proposed by Lorite et al. (2017) , in which erythrosine B food dye printed on PLA was applied in a microfluidic device in conjunction with a frozen solvent. As soon as the temperature exceeds the melting point, the transparent solvent flows and dissolves the dye producing red staining of the device.
Other potentially viable food packaging sensors include electrical or RFID/NFC based ( Lorite et al., 2017 ; Barandun et al., 2019 ) and electrochemical devices ( Oliveira et al., 2013 ). Lorite et al. (2017) developed further their solvent melting point based colorimetric temperature sensor by using an electrically conductive film of carbon nanotubes being a part of an RFID tag. When the temperature reached the melting point of the solvent, it flowed through a capillary toward the nanotube film, soaked it and increased its resistance detected by the RFID reader. Very recently, an interesting and simple resistive chemical sensor for water-soluble gasses was proposed by Barandun et al. (2019) on cellulose substrates. As water is always present on the surface of the hygroscopic cellulose, when it is exposed to water-soluble gas analytes, the surface conductivity increases depending on the chemistry and concentration of the interacting moiety, which can be monitored by electrical measurements using carbon electrodes printed on the surface. The devices were highly sensitive to ammonia (down to 200 ppb) among the gasses tested (TMA, H 2 S, CO 2 , and CO) and were feasible for monitoring the headspace of meat and fish food packages. The sensors could be integrated into RFID tags and read by an NFC enabled smartphone ( Figure 6 ).
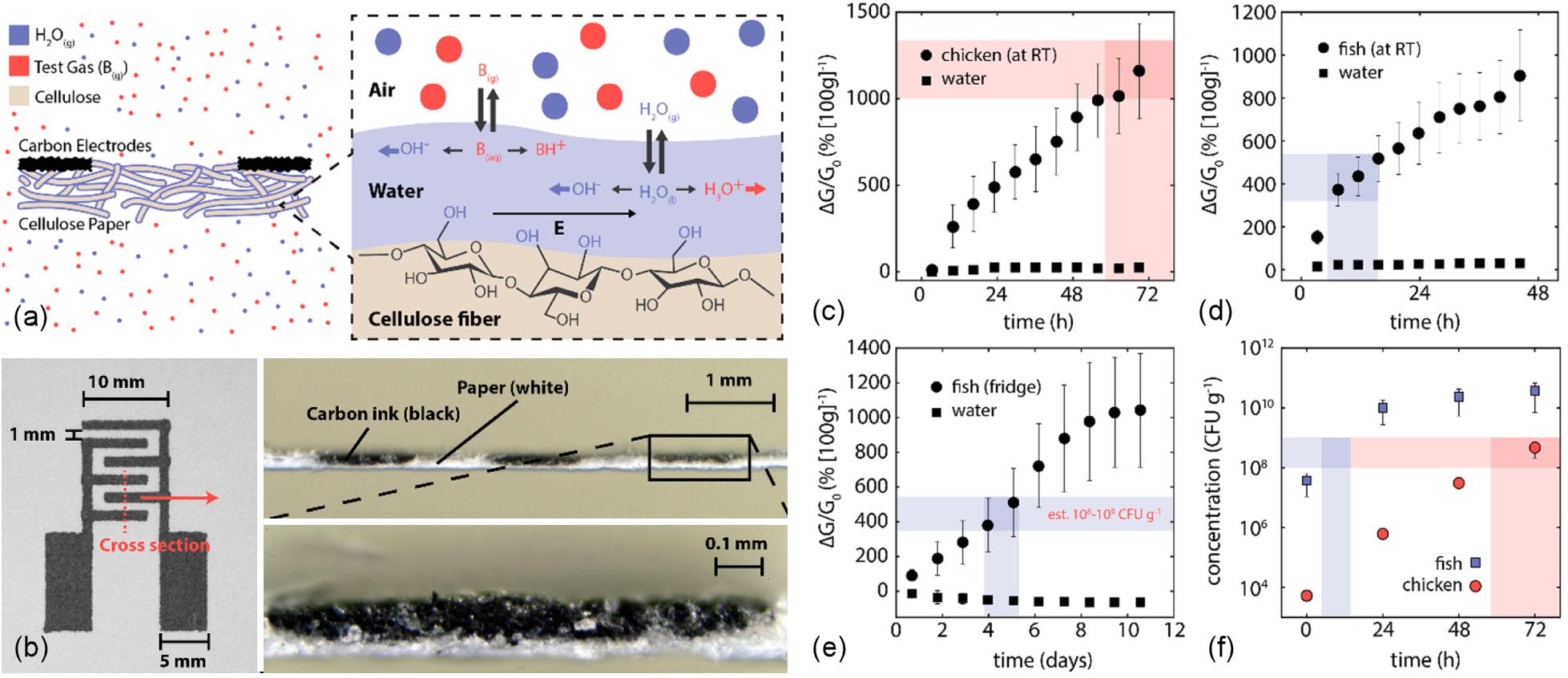
Figure 6. (A) Schematic diagram displaying the equilibrium of surface adsorbed water and vapor in the gas phase. Absorbed and then dissociated base increases the concentration of mobile ions in the liquid. (B) Optical images of the printed carbon electrodes on paper (top and cross-sectional views). Relative change of sensor conductance measured for decaying (C) meat and (D) fish at room temperature, and (E) fish at 4°C. The red and gray bands in the graphs correspond to the healthy limit of microbial contamination determined by microbial cultures displayed in panel (F) . [Reproduced from Barandun et al. (2019) with permission from American Chemical Society].
The price of the bio-based food packaging sensors is directly connected to the price of foodstuff as many of them originate from natural sources and are edible. For example, the price of curcumin is around 1 380 €/kg (February 2020) or for the wild blueberries the purchase price is typically between 2.5 and 4 €/kg (in Finland) but may rise up to 6–8 €/kg if the availability is limited due to dry summer, for example like in Finland in 2019. However, as pointed out earlier, edible food should not be used as raw material, but rather side streams should be valorized. Luckily the anthocyanin content is reasonable high in pomaces and seeds, so in principle agro-food waste can be used as source for sensor material lowering the price. If we compare the prices of food to prices of typical gas sensor materials, such as titanium dioxide with bulk price around 2–3 €/kg (2016–2017, Industrial Minerals), the anthocyanin source are competitive, especially because colorimetric sensors do not require complex electronics for the output and the waste management can be expected to be cheaper.
Bio-based smart food packaging will be one answer to the global challenges related to the desperate quest for carbon neutrality, food saving and safety, as well as for renewable materials and technologies. In this review, we have collected the contemporary literature on three key components of bio-based smart materials including (i) the packaging materials themselves responsible for providing a safe envelope for the products, (ii) advanced coatings and additives to help preserving foodstuff as well as (iii) renewable sensor materials with enabling technologies that can detect the quality of foods and are potentially feasible for industrial scale-up. Although the corresponding fields of scientific research on bio-based and renewable materials with robust production technologies are becoming more and more relevant today, it is clear that careful life cycle, economic and even user perception analyses have to be made to assess the real environmental and socioeconomic impact of each potentially viable solution.
Author Contributions
NH and KK contributed to the conception and design of the review. NH wrote the first draft of the manuscript. PP contributed with the literature search, illustrations, and writing of the manuscript. AB, CF, RN, and GS wrote sections of the manuscript. All authors contributed to manuscript revision, read and approved the submitted version.
The authors kindly acknowledge financial support from the EU Horizon 2020 – BBI JU under agreement no. 792261 NewPack, and EU Interreg Nord – Lapin liitto under agreement no. 20201468 Flexible transparent conductive films as electrodes.
Conflict of Interest
The authors declare that the research was conducted in the absence of any commercial or financial relationships that could be construed as a potential conflict of interest.
Abbreviations
Bio-PA, bio-polyamide; Bio-PBS, bio-polybutylene succinate; Bio-PE, bio-polyethylene; Bio-PET, polyethylene terephthalate containing bio-based materials; Bio-PP, bio-polypropylene; CA, cellulose acetate; FDCA, 2,5-furandicarboxylic acid; HDPE, high-density polyethylene; HIPS, high impact polystyrene; LDPE, low-density polyethylene; LLDPE, linear low-density polyethylene; MEG, mono-ethylene glycol; NFC, near-field communication; PA, polyamide; PBAT, poly(butylene adipate-co-terephthalate); PBS, polybutylene succinate; PBSA, polybutylene succinate adipate; PBST, poly(butylene succinate-co-terephthalate); PCL, polycaprolactone; PE, polyethylene; PEA, polyesteramide; PEF, polyethylene furanoate; PES, polyethersulfone; PET, polyethylene terephthalate; PHA, polyhydroxyalkanoate; PHB, polyhydroxybutyrate; PHBV, poly(hydroxybutyrate-co-valerate); PLA, polylactic acid; PP, polypropylene; PS, polystyrene; PTT, polytrimethylene terephthalate; PVA, polyvinyl alcohol; PVC, polyvinyl chloride; PVdC, polyvinylidene chloride; PVOH, polyvinyl alcohol; RFID, radio frequency identification; TA, terephthalic acid; TPS, thermoplastic starch; UV, ultra violet.
- ^ Amazon.co.uk
Abolghasemi, M. M., Sobhi, M., and Piryaei, M. (2016). Preparation of a novel green optical pH sensor based on immobilization of red grape extract on bioorganic agarose membrane. Sens. Actuat. B 224, 391–395. doi: 10.1016/j.snb.2015.10.038
CrossRef Full Text | Google Scholar
Ackermann, J.-U., Müller, S., Lösche, A., Bley, T., and Babel, W. (1995). Methylobacterium rhodesianum cells tend to double the DNA content under growth limitations and accumulate PHB. J. Biotechnol. 39, 9–20. doi: 10.1016/0168-1656(94)00138-3
Ahmad, N. A., Heng, L. Y., Salam, F., Zaid, M. H. M., and Hanifah, S. A. (2019). A colorimetric pH sensor based on Clitoria sp and Brassica sp for monitoring of food spoilage using chromametry. Sensors 19, :4813. doi: 10.3390/s19214813
PubMed Abstract | CrossRef Full Text | Google Scholar
Ahmed, I., Lin, H., Zou, L., Li, Z., Brody, A. L., Qazi, I. M., et al. (2018). An overview of smart packaging technologies for monitoring safety and quality of meat and meat products. Packag. Technol. Sci. 31, 449–471. doi: 10.1002/pts.2380
Aider, M. (2010). Chitosan application for active bio-based films production and potential in the food industry: review. LWT— Food Sci. Technol. 43, 837–842. doi: 10.1016/j.lwt.2010.01.021
Arroyo, B. J., Santos, A. P., de Melo, E. D. A., Campos, A., Lins, L., and Boyano-Orozco, L. C. (2019). “Chapter 8 – Bioactive compounds and their potential use as ingredients for food and its application in food packaging,” in Bioactive Compounds: Health Benefits and Potential Applications , ed. M. R. S. Campos (Sawston: Woodhead Publishing), 143–156. doi: 10.1016/B978-0-12-814774-0.00008-6
Avérous, L. (2008). “Chapter 21 – Polylactic acid: synthesis, properties and applications,” in Monomers, Polymers and Composites from Renewable Resources , eds M. N. Belgacem and A. Gandini (Oxford: Elsevier), 433–450. doi: 10.1016/B978-0-08-045316-3.00021-1
Badia-Melis, R., Mc Carthy, U., Ruiz-Carcia, L., Carcia-Hierro, J., and Robla Villalba, J. I. (2018). New trends in cold chain monitoring applications – a review. Food Control 86, 170–182. doi: 10.1016/j.foodcont.2017.11.022
Barandun, G., Soprani, M., Naficy, S., Grell, M., Kasimatis, M., Chiu, K. L., et al. (2019). Cellulose fibers enable near-zero-cost electrical sensing of water-soluble gases. ACS Sens. 4, 1662–1669. doi: 10.1021/acssensors.9b00555
Bassani, A., Montes, S., Jubete, E., Palenzuela, J., Sanjuan, A. P., and Spigno, G. (2019). Incorporation of waste orange peels extracts into PLA films. Chem. Eng. Trans. 74, 1063–1068. doi: 10.3303/CET1974178
Bibi, F., Guillaume, C., Gontard, N., and Sorli, B. (2017). Wheat gluten, a bio-polymer to monitor carbon dioxide in food packaging: electric and dielectric characterization. Sens. Actuat. B 250, 76–84. doi: 10.1016/j.snb.2017.03.164
Branigan, T. (2008). Chinese Figures Show Fivefold Rise in Babies Sick from Contaminated Milk, The Guardian, 8 December. Available online at: https://www.theguardian.com/world/2008/dec/02/china (accessed December 12, 2019) Google Scholar
Brockgreitens, J., and Abbas, A. (2016). Responsive food packaging: recent progress and technological prospects. Compr. Rev. Food Sci. Food Safe. 15, 3–15. doi: 10.1111/1541-4337.12174
Bulushi, I. A., Poole, S., Deeth, H. C., and Dykes, G. A. (2009). Biogenic amines in fish: roles in intoxication, spoilage, and nitrosamine formation - – a review. Crit. Rev. Food Sci. 49, 369–377. doi: 10.1080/10408390802067514
Chayavanich, K., Thiraphibundet, P., and Imyim, A. (2020). Biocompatible film sensors containing red radish extract for meat spoilage observation. Spectrochim. Acta A. 226, 117601. doi: 10.1016/j.saa.2019.117601
Chen, G. Q., and Patel, M. K. (2012). Plastics derived from biological sources: present and future: a technical and environmental review. Chem. Rev. 112, 2082–2099. doi: 10.1021/cr200162d
Chiellini, E., Corti, A., D’Antone, S., and Solaro, B. (2003). Biodegradation of poly (vinyl alcohol) based materials. Prog. Polym. Sci. 28, 963–1014. doi: 10.1016/S0079-6700(02)00149-1
Choi, I., Lee, Y. J., Lacroix, M., and Han, J. (2017). Intelligent pH indicator film composed of agar/potato starch and anthocyanin extracts from purple sweet potato. Food Chem. 218, 122–128. doi: 10.1016/j.foodchem.2016.09.050
da Silva, C. K., da Silveira Mastrantonia, D. J., Costa, J. A. V., and de Morais, M. G. (2019). Innovative pH sensors developed from ultrafine fibers containing açaí ( Euterpe oleracea ) extract. Food Chem. 294, 397–404. doi: 10.1016/j.foodchem.2019.05.059
Dudnyk, I., Janeèek, E.-R., Vaucher-Joset, J., and Stellacci, F. (2018). Edible sensors for meat and seafood freshness. Sens. Actuat. B 259, 1108–1112. doi: 10.1016/j.snb.2017.12.057
European Bioplastics e.V (2019). What are Bioplastics?. Available online at: https://www.european-bioplastics.org/bioplastics/ (accessed December 13, 2019) Google Scholar
European Bioplastics e.V (2020). Bioplastics Market Data. Available online at: https://www.european-bioplastics.org/market/ (accessed January 20, 2020) Google Scholar
Europol (2015). Operation Opson IV – Case Stories. Available online at: https://www.europol.europa.eu/publications-documents/operation-opson-iv-case-stories (accessed December 12, 2019) Google Scholar
Ezati, P., Tajik, H., Moradi, M., and Molaei, R. (2019). Intelligent pH-sensitive indicator based on starch-cellulose and alizarin dye to track freshness of rainbow trout fillet. Int. J. Biol. Macromol. 132, 157–165. doi: 10.1016/j.ijbiomac.2019.03.173
Food and Agriculture Organization of the United Nations (2019a). Food Loss and Food Waste, The United Nations. Available online at: http://www.fao.org/food-loss-and-food-waste/en/ (accessed December 12, 2019) Google Scholar
Food and Agriculture Organization of the United Nations. (2019b). Sustainable Development Goals, 2 Zero Hunger, The United Nations. Available online at: http://www.fao.org/sustainable-development-goals/goals/goal-2/en/ (accessed December 12, 2019) Google Scholar
Fuertes, G., Soto, I., Carrasco, R., Vargas, M., Sabattin, J., and Lagos, C. (2016). Intelligent packaging systems: sensors and nanosensors to monitor food quality and safety. J. Sensors 2016, :4046061. doi: 10.1155/2016/4046061
Galstyan, V., Bhandari, M. P., Sberveglieri, V., Sberveglieri, G., and Comini, E. (2018). Metal oxide nanostructures in food applications: quality control and packaging. Chemosensors 6, :16. doi: 10.3390/chemosensors6020016
Gamage, G. R., Park, H. J., and Kim, K. M. (2009). Effectiveness of antimicrobial coated oriented polypropylene/polyethylene films in sprout packaging. Food Res. Int. 42, 832–839. doi: 10.1016/j.foodres.2009.03.012
Geueke, B. (2014). Dossier – Bioplastics as Food Contact Materials, Food Packaging Forum. doi: 10.5281/zenodo.33517 Available online at: https://www.foodpackagingforum.org/fpf-2016/wp-content/uploads/2015/11/FPF_Dossier06_Bioplastics.pdf (accessed December 13, 2019)
Ghaani, M., Cozzolino, C. A., Castelli, G., and Farris, S. (2016). An overview of the intelligent packaging technologies in the food sector. Trends Food Sci. Tech. 51, 1–11. doi: 10.1016/j.tifs.2016.02.008
Gómez-Estaca, J., López-de-Dicastillo, C., Hernández-Muñoz, P., Catalá, R., and Gavara, R. (2014). Advances in antioxidant active food packaging. Trends Food Sci. Tech. 35, 42–51. doi: 10.1016/j.tifs.2013.10.008
Grayhurst, P., and Girling, P. J. (2011). “Packaging of food in glass containers,” in Food and Beverage Packaging Technology , 2nd Edn, eds R. Coles and M. Kirwan (Hoboken, NJ: Blackwell Publishing Ltd), 137–156. doi: 10.1002/9781444392180.ch6
Ha, J. U., Kim, Y. M., and Lee, D. S. (2001). Multilayered antimicrobial polyethylene films applied to the packaging of ground beef. Packag. Technol. Sci. 14, 55–62. doi: 10.1002/pts.537
Holley, R. A., and Patel, D. (2005). Improvement in shelf-life and safety of perishable foods by plant essential oils and smoke antimicrobials. Food Microbiol. 22, 273–292. doi: 10.1016/j.fm.2004.08.006
Jamieson, A. J., Brooks, L. S. R., Reid, W. D. K., Piertney, S. B., Narayanaswamy, B. E., and Linley, T. D. (2019). Microplastics and synthetic particles ingested by deep-sea amphipods in six of the deepest marine ecosystems on Earth. Roy. Soc. Open Sci. 6, :180667. doi: 10.1098/rsos.180667
Jo, H.-J., Park, K.-M., Na, J. H., Min, S. C., Park, K. H., Chang, P.-S., et al. (2015). Development of anti-insect food packaging film containing a polyvinyl alcohol and cinnamon oil emulsion at a pilot plant scale. J. Stored Prod. Res. 61, 114–118. doi: 10.1016/j.jspr.2015.01.005
Kechichian, V., Ditchfield, C., Veiga-Santos, P., and Tadini, C. C. (2010). Natural antimicrobial ingredients incorporated in biodegradable films based on cassava starch. LWT— Food Sci. Technol. 43, 1088–1094. doi: 10.1016/j.lwt.2010.02.014
Kirchner, N., Zedler, L., Mayerhöfer, T. G., and Mohr, G. J. (2006). Functional liquid crystal films selectively recognize amine vapours and simultaneously change their colour. Chem. Commun. 14, 1512–1514. doi: 10.1039/B517768E
Kirwan, M. J. (2011). “Paper and paperboard packaging,” in Food and Beverage Packaging Technology , 2nd Edn, eds R. Coles and M. Kirwan (Hoboken, NJ: Blackwell Publishing Ltd), 213–250. doi: 10.1002/9781444392180.ch8
Kong, J.-M., Chia, L.-S., Goh, N.-K., Chia, T.-F., and Brouillard, R. (2003). Analysis and biological activities of anthocyanins. Phytochemistry 64, 923–933. doi: 10.1016/S0031-9422(03)00438-2
Koopman, F., Wierckx, N., de Winde, J. H., and Ruijssenaars, H. J. (2010a). Efficient whole-cell biotransformation of 5-(hydroxymethyl)furfural Into FDCA, 2,5-furandicarboxylic acid. Bioresour. Technol. 101, 6291–6296. doi: 10.1016/j.biortech.2010.03.050
Koopman, F., Wierckx, N., de Winde, J. H., and Ruijssenaars, H. J. (2010b). Identification and characterization of the furfural and 5-(hydroxymethyl)furfural degradation pathways of Cupriavidus basilensis HMF14. Proc. Natl. Acad. Sci. U.S.A. 107, 4919–4924. doi: 10.1073/pnas.0913039107
Kraus, F. J., and Tarulis, G. J. (2009). in Cans, Steel, Encyclopedia of Packaging Technology , 3rd Edn, ed. K. L. Yam (Hoboken, NJ: Wiley), 205–216.
Google Scholar
Kuorwel, K. K., Cran, M. J., Orbell, J. D., Buddhadasa, S., and Bigger, S. W. (2015). Review of mechanical properties, migration, and potential applications in active food packaging systems containing nanoclays and nanosilver. Compr. Rev. Food Sci. Food Safe.. 14, 411–430. doi: 10.1111/1541-4337.12139
Kurek, M., Garofuliæ, I. E., Bakiæ, M. T., Šèetar, M., Uzelac, V. D., and Galiæ, K. (2018). Development and evaluation of a novel antioxidant and pH indicator film based on chitosan and food waste sources of antioxidants. Food Hydrocolloid. 84, 238–246. doi: 10.1016/j.foodhyd.2018.05.050
Kurek, M., Hlupiæ, L., Šèetar, M., Bosiljkov, T., and Galiæ, K. (2019). Comparison of two pH responsive color changing bio−based films containing wasted fruit pomace as a source of colorants. J. Food Sci. 84, 2490–2498. doi: 10.1111/1750-3841.14716
Kuswandi, B., Jayus, Larasati, T. S., Abdullah, A., and Heng, L. Y. (2012). Real-time monitoring of shrimp spoilage using on-package sticker sensor based on natural dye of curcumin. Food Anal. Methods 5, 881–889. doi: 10.1007/s12161-011-9326-x
Kuswandi, B., Wicaksono, Y., Jayus, Abdullah, A., Heng, L. Y., and Ahmad, M. (2011). Smart packaging: sensors for monitoring of food quality and safety. Sens. & Instrum. rumen. Food Qual. Safe. 5, 137–146. doi: 10.1007/s11694-011-9120-x
Lei, J., Yang, L., Zhan, Y., Wang, Y., Ye, T., Li, Y., et al. (2014). Plasma treated polyethylene terephthalate/polypropylene films assembled with chitosan and various preservatives for antimicrobial food packaging. Colloids. Surf. B: Biointerfaces. 114, 60–66. doi: 10.1016/j.colsurfb.2013.09.052
Liang, T., Sun, G., Cao, L., Li, J., and Wang, L. (2019). A pH and NH3 sensing intelligent film based on Artemisia sphaerocephala Krasch. gum and red cabbage anthocyanins anchored by carboxymethyl cellulose sodium added as a host complex. Food Hydrocoll.loid 87, 858–868. doi: 10.1016/j.foodhyd.2018.08.028
Liang, T., and Wang, L. (2018). A pH-sensing film from tamarind seed polysaccharide with litmus lichen extract as an indicator. Polymers 10, :13. doi: 10.3390/polym10010013
Lin, J.-F., Kukkola, J., Sipola, T., Raut, D., Samikannu, A., Mikkola, J.-P., et al. (2015). Trifluoroacetylazobenzene for optical and electrochemical detection of amines. J. Mater. Chem. A 3, 4687–4694. doi: 10.1039/C4TA05358C
Liu, B., Xu, H., Zhao, H., Liu, W., Zhao, L., and Li, Y. (2017). Preparation and characterization of intelligent starch/PVA films for simultaneous colorimetric indication and antimicrobial activity for food packaging applications. Carbohyd. Polym. 157, 842–849. doi: 10.1016/j.carbpol.2016.10.067
Liu, J., Wang, H., Wang, P., Guo, M., Jiang, S., Li, X., et al. (2018). Films based on κ-carrageenan incorporated with curcumin for freshness monitoring. Food Hydrocoll.oid. 83, 134–142. doi: 10.1016/j.foodhyd.2018.05.012
Lorite, G. S., Selkälä, T., Sipola, T., Palenzuela, J., Jubete, E., Viñuales, A., et al. (2017). Novel, smart and RFID assisted critical temperature indicator for supply chain monitoring. J. Food Eng. 193, 20–28. doi: 10.1016/j.jfoodeng.2016.06.016
Luchese, C. L., Pavoni, J. M. F., Spada, J. C., and Tessaro, I. C. (2019). Influence of blueberry and jaboticaba agroindustrial residue particle size on color change of corn starch based films submitted to different pH values solutions. J. Renew. Mater. 7(3), 235–243, 2019. doi: 10.32604/jrm.2019.00033
Luchese, C. L., Sperotto, N., Spada, J. C., and Tessaro, I. C. (2017). Effect of blueberry agro-industrial waste addition to corn starch-based films for the production of a pH-indicator film. Int. J. Biol. Macromol. 104, 11–18. doi: 10.1016/j.ijbiomac.2017.05.149
Ma, Q., Du, L., and Wang, L. (2017a). Tara gum/polyvinyl alcohol-based colorimetric NH3 indicator films incorporating curcumin for intelligent packaging. Sens. Actuat. B 244, 759–766. doi: 10.1016/j.snb.2017.01.035
Ma, Q., Liang, T., Cao, L., and Wang, L. (2018). Intelligent poly (vinyl alcohol)-chitosan nanoparticles-mulberry extracts films capable of monitoring pH variations. Int. J. Biol. Macromol. 108, 576–584. doi: 10.1016/j.ijbiomac.2017.12.049
Ma, Q., Ren, Y., Gu, Z., and Wang, L. (2017b). Developing an intelligent film containing Vitis amurensis husk extracts: the effects of pH value of the film-forming solution. J. Clean. Prod. 166, 851–859. doi: 10.1016/j.jclepro.2017.08.099
Ma, Y., Li, L., and Wang, Y. (2018). Development of PLA-PHB-based biodegradable active packaging and its application to salmon. Packag. Technol. Sci. 31, 739–746. doi: 10.1002/pts.2408
Machado, P. G., Walter, A., and Cunha, M. (2016). Bio−based propylene production in a sugarcane biorefinery: a techno−economic evaluation for Brazilian conditions. Biofuel. Bioprod. Bior. 10, 623–633. doi: 10.1002/bbb.1674
Maftoonazad, N., and Ramaswamy, H. (2019). Design and testing of an electrospun nanofiber mat as a pH biosensor and monitor the pH associated quality in fresh date fruit (Rutab). Polym. Test. 75, 76–84. doi: 10.1016/j.polymertesting.2019.01.011
Majdinasab, M., Hosseini, S. M. H., Sepidname, M., Negahdarifar, M., and Li, P. (2018). Development of a novel colorimetric sensor based on alginate beads for monitoring rainbow trout spoilage. J. Food Sci. Technol. 55, 1695–1704. doi: 10.1007/s13197-018-3082-5
Manso, S., Becerril, R., Nerín, C., and Gómez-Lus, R. (2015). Influence of pH and temperature variations on vapor phase action of an antifungal food packaging against five mold strains. Food Contr. 47, 20–26. doi: 10.1016/j.foodcont.2014.06.014
Medina-Jaramillo, C., Ochoa-Yepes, O., Bernal, C., and Famá, L. (2017). Active and smart biodegradable packaging based on starch and natural extracts. Carbohyd. Polym. 176, 187–194. doi: 10.1016/j.carbpol.2017.08.079
Mlalila, N., Hilonga, A., Swai, H., Devlieghere, F., and Ragaert, P. (2018). Antimicrobial packaging based on starch, poly (3-hydroxybutyrate) and poly (lactic-co-glycolide) materials and application challenges. Trends Food Sci. Tech. 74, 1–11. doi: 10.1016/j.tifs.2018.01.015
Mohr, G. J. (2004a). Chromo- and fluororeactands: indicators for detection of neutral analytesby using reversible covalent-bond chemistry. Chem. Eur. J. 10, 1082–1090. doi: 10.1002/chem.200305524
Mohr, G. J. (2004b). Tailoring the sensitivity and spectral properties of a chromoreactand for the detection of amines and alcohols. Anal. Chim. Acta 508, 233–237. doi: 10.1016/j.aca.2003.12.005
Muncke, J. (2012). Food Packaging Materials, Food Packaging Forum. Available online at: https://www.foodpackagingforum.org/food-packaging-health/food-packaging-materials (accessed December 13, 2019) Google Scholar
Muriel-Galet, V., Cerisuelo, J. P., López-Carballo, G., Aucejo, S., Gavara, R., and Hernández-Muñoz, P. (2013). Evaluation of EVOH-coated PP films with oregano essential oil and citral to improve the shelf-life of packaged salad. Food Control 30, 137–143. doi: 10.1016/j.foodcont.2012.06.032
Musso, Y. S., Salgado, P. R., and Mauri, A. N. (2017). Smart edible films based on gelatin and curcumin. Food Hydrocoll.oids 66, 8–15. doi: 10.1016/j.foodhyd.2016.11.007
Musso, Y. S., Salgado, P. R., and Mauri, A. N. (2019). Smart gelatin films prepared using red cabbage ( Brassica oleracea L.) extracts as solvent. Food Hydrocoll.oid. 89, 674–681. doi: 10.1016/j.foodhyd.2018.11.036
Mustafa, F., and Andreescu, S. (2018). Chemical and biological sensors for food-quality monitoring and smart packaging. Foods 7, :168. doi: 10.3390/foods7100168
Narwade, V. N., Anjum, S. R., Kokol, V., and Khairnar, R. S. (2019). Ammonia-sensing ability of differently structured hydroxyapatite blended cellulose nanofibril composite films. Cellulose 26, 3325–3337. doi: 10.1007/s10570-019-02299-y
Niaounakis, M. (2013). Biopolymers: Reuse, Recycling and Disposal , 1st Edn. Amsterdam: Elsevier. doi: 10.1007/s10570-019-02299-y
Nquyen, L. H., Naficy, S., McConchie, R., Dehghani, F., and Chandrawati, R. (2019). Polydiacetylene-based sensors to detect food spoilage at low temperatures. J. Mater. Chem. C 7, 1919–1926. doi: 10.1039/c8tc05534c
Oldring, P. K. T., and Nehring, U. (2007). Packaging Materials; 7. Metal Packaging for Foodstuffs, The International Life Sciences Institute. Available online at: < https://www.pac.gr/bcm/uploads/7-metal-packaging-for-foodstuffs.pdf (accessed 13 December 13, 2019) Google Scholar
Oliveira, T. M., Fátima Barroso, M., Morais, S., de Lima-Neto, P., Correia, A. N., Oliveira, M. B., et al. (2013). Biosensor based on multi-walled carbon nanotubes paste electrode modified with laccase for pirimicarb pesticide quantification. Talanta 106, 137–143. doi: 10.1016/j.talanta.2012.12.017
Peelman, N., Ragaert, P., De Meulenaer, B., Adons, D., Peeters, R., Cardon, L., et al. (2013). Application of bioplastics for food packaging. Trends Food Sci. Tech. 32, 128–141. doi: 10.1016/j.tifs.2013.06.003
Pénicaud, C., Achir, N., Dhuique-Mayer, C., Dornier, M., and Bohoun, P. (2011). Degradation of β-carotene during fruit and vegetable processing or storage: reaction mechanisms and kinetic aspects: a review. Fruits 66, 417–440. doi: 10.1051/fruits/2011058
Pereira, P. F., and Andrade, C. T. (2017). Optimized pH-responsive film based on a eutectic mixture-plasticized chitosan. Carbohyd. Polym. 165, 238–246. doi: 10.1016/j.carbpol.2017.02.047
Plackett, D. V., Holm, V. K., Johansen, P., Ndoni, S., Nielsen, P. V., Sipilainen-Malm, T., et al. (2006). Characterization of L-potylactide and L-polylactide-polycaprolactone co-polymer films for use in cheese-packaging applications. Packag. Technol. Sci. 19, 1–24. doi: 10.1002/pts.704
PlasticsEurope. (2019a). Plastics – The Facts 2019. Available online at: https://www.plasticseurope.org/application/files/1115/7236/4388/FINAL_web_version_Plastics_the_facts2019_14102019.pdf (accessed December 13, 2019) Google Scholar
PlasticsEurope. (2019b). Plastics in Packaging. Available online at: https://www.plasticseurope.org/en/about-plastics/packaging (accessed December 13, 2019) Google Scholar
Pourjahaver, S., Almasi, H., Meshkini, S., Pirsa, S., and Parandi, E. (2017). Development of a colorimetric pH indicator based on bacterial cellulose nanofibers and red cabbage ( Brassica oleraceae ) extract. Carbohyd. Polym. 156, 193–201. doi: 10.1016/j.carbpol.2016.09.027
Prester, L. (2011). Biogenic amines in fish, fish products and shellfish: a review. Food Addit. Contam. A 28, 1547–1560. doi: 10.1080/19440049.2011.600728
Prietto, L., Mirapalhete, T. C., Pinto, V. Z., Hoffmann, J. F., Vanier, N. L., Lim, L.-T., et al. (2017). pH-sensitive films containing anthocyanins extracted from black bean seed coat and red cabbage. LWT- Food Sci. Technol. 80, 492–500. doi: 10.1016/j.lwt.2017.03.006
Rani, D. N., and Abraham, T. E. (2006). Kinetic study of a purified anionic peroxidase isolated from Eupatorium odoratum and its novel application as time temperature indicator for food materials. J. Food Eng. 77, 594–600. doi: 10.1016/j.jfoodeng.2005.07.018
Reinert, S., and Mohr, G. J. (2008). Chemosensor for the optical detection of aliphatic amines and diamines. Chem. Commun 19, 2272–2274. doi: 10.1039/B717796H
Reingardt, T., and Nieder, N. F. (2009). in Cans, Aluminium, Encyclopedia of Packaging Technology , 3rd Edn, ed. K. L. Yam (Hoboken, NJ: Wiley), 193–195.
Robertson, G. L. (2012). Food Packaging: Principles and Practice , 3rd Edn. Boca Raton, FL: CRC Press.
Saliu, F., and Pergola, R. D. (2018). Carbon dioxide colorimetric indicators for food packaging applications: applicability of anthocyanin and poly-lysine mixtures. Sens. Actuat. B 258, 1117–1124. doi: 10.1016/j.snb.2017.12.007
Sarfraz, J., Määttänen, A., Ihalainen, P., Keppeler, M., Lindén, M., and Peltonen, J. (2012). Printed copper acetate based H2S sensor on paper substrate. Sens. Actuat. B 173, 868–873. doi: 10.1016/j.snb.2012.08.008
Schut, J. H. (2016). Pioneering Sustainability; biopolymer applications are expanding, in fitsand starts. Plast. ics Eng.ineering 72, 6–13. doi: 10.1002/j.1941-9635.2016.tb01490.x
Šešlija, S., Nešiæ, A., Škoriæ, M. L., Krušiæ, M. K., Santagata, G., and Malinconico, M. (2018). Pectin/carboxymethylcellulose films as a potential food packaging material. Macromol. Symp. 378, :1600163. doi: 10.1002/masy.201600163
Seydim, A. C., and Sarikus, G. (2006). Antimicrobial activity of whey protein based edible films incorporated with oregano, rosemary and garlic essential oils. Food Res. Intl. 39, 639–644. doi: 10.1016/j.foodres.2006.01.013
Shukla, V., Kandeepan, G., Vishnuraj, M. R., and Soni, A. (2016). Anthocyanins based indicator sensor for intelligent packaging application. Agric. Res 5, 205–209. doi: 10.1007/s40003-016-0211-0
Silva, E. B., Ferreira, P. V., Chretien, J. B., Miranca, J. I. S., Pinho, H. A., Timbó, ÁP., et al. (2017). Green optical dissolved oxygen sensor based on a chlorophyll–zinc complex extracted from the plant Brassica oleracea L. Appl. Optics 36, 9951–9956. doi: 10.1364/AO.56.009951
Silva-Pereira, M. C., Teixeira, J. A., Pereira-Júnior, V. A., and Stefani, R. (2015). Chitosan/corn starch blend films with extract from Brassica oleraceae (red cabbage) as a visual indicator of fish deterioration. LWT— Food Sci. Technol. 61, 258–262. doi: 10.1016/j.lwt.2014.11.041
The United Nations. (2019). World Population Prospects 2019: Highlights, Department of Economic and Social Affairs. Available online at: https://www.un.org/development/desa/publications/world-population-prospects-2019-highlights.html (accessed December 18, 2019) Google Scholar
Torlak, E., and Nizamlioğlu, M. (2011). Antimicrobial effectiveness of chitosan-essential oil coated plastic films against foodborne pathogens. J. Plast. Film Sheet. 27, 235–248. doi: 10.1177/8756087911407391
Valderrama Solano, A. C., and de Rojas Gante, C. (2012). Two different processes to obtain antimicrobial packaging containing natural oils. Food Bioprocess. Tech. 5, 2522–2528. doi: 10.1007/s11947-011-0626-3
van Crevel, R. (2016). Bio-Based Food Packaging in Sustainable Development, Food and Agriculture Organization of the United Nations. Available online at: http://www.fao.org/forestry/45849-023667e93ce5f79f4df3c74688c2067cc.pdf (accessed 17 December 2019) Google Scholar
van den Broek, L. A. M., Knoop, R. J. I., Kappen, F. H. J., and Boeriu, C. G. (2015). Chitosan films and blends for packaging material. Carbohydr. Polym. 116, 237–242. doi: 10.1016/j.carbpol.2014.07.039
van den Oever, M., Molenveld, K., van der Zee, M., and Bos, H. (2017). Bio-Based and Biodegradable Plastics : Facts and Figures : Focus on Food Packaging in the Netherlands , Wageningen Food & Biobased Research No. 1722. Wageningen: Food & & Biobased Research, doi: 10.18174/408350
Vanderroost, M., Ragaert, P., Devlieghere, F., and De Meulenaer, B. (2014). Intelligent food packaging: the next generation. Trends Food Sci. Tech. 39, 47–62. doi: 10.1016/j.tifs.2014.06.009
Vermeiren, L., Devlieghere, F., and Debevere, J. (2002). Effectiveness of some recent antimicrobial packaging concepts. Food Addit. Contam. 19, 163–171. doi: 10.1080/02652030110104852
Wang, L. F., and Rhim, J. W. (2016). Grapefruit seed extract incorporated antimicrobial LDPE and PLA films: effect of type of polymer matrix. LWT- Food Sci. Technol. 74, 338–345. doi: 10.1016/j.lwt.2016.07.066
Weinmann, R. C., and Cotton, R. A. (1958). United States Patent Office Patent Number 2,849,319. Available online at: https://patentimages.storage.googleapis.com/da/c8/a2/5bacc8e97bef21/US2849319.pdf (accessed December 15, 2019).
World Economic Forum, Ellen MacArthur Foundation and McKinsey Company. (2016). The New Plastics Economy — Rethinking the Future of Plastics. Available online at: https://www.ellenmacarthurfoundation.org/publications/the-new-plastics-economy-rethinking-the-future-of-plastics (accessed December 13, 2019) Google Scholar
World Health Organization. (2019). Food Safety, The United Nations. Available online at: https://www.who.int/news-room/fact-sheets/detail/food-safety (accessed 12 December 12, 2019) Google Scholar
Wrona, M., Nerín, C., Alfonso, M. J., and Caballero, M. Á (2017). Antioxidant packaging with encapsulated green tea for fresh minced meat, Innov. Food Sci. Emerg. 41, 307–313. doi: 10.1016/j.ifset.2017.04.001
Xiaowei, H., Xiaobo, Z., Jiewen, Z., Jiyong, S., Zhihua, L., and Tingting, S. (2015). Monitoring the biogenic amines in Chinese traditional salted pork in jelly (Yao−meat) by colorimetric sensor array based on nine natural pigments. Int. J. Food Sci. Technol. 50, 203–209. doi: 10.1111/ijfs.12620
Yam, K. L., Takhistrov, P. T., and Miltz, J. (2005). Intelligent packaging: concepts and applications. J. Food. Sci. 70, R1–R10. doi: 10.1111/j.1365-2621.2005.tb09052.x
Yan, S., Huawei, C., Limin, Z., Fazheng, R., Luda, Z., and Hengtao, Z. (2008). Development and characterization of a new amylase type time–temperature indicator. Food Control 19, 315–319. doi: 10.1016/j.foodcont.2007.04.012
Yanxiao, L., Xiao-bo, Z., Xiao-wei, H., Ji-yong, S., Jie-wen, Z., Holmes, M., et al. (2015). A new room temperature gas sensor based on pigment-sensitized TiO2 thin film for amines determination. Biosens. Bioelectron. 67, 35–41. doi: 10.1016/j.bios.2014.05.040
Ye, M., Neetoo, H., and Chen, H. (2008a). Control of Listeria monocytogenes on ham steaks by antimicrobials incorporated into chitosan-coated plastic films. Food Microbiol. 25, 260–268. doi: 10.1016/j.fm.2007.10.014
Ye, M., Neetoo, H., and Chen, H. (2008b). Effectiveness of chitosan-coated plastic films incorporating antimicrobials in inhibition of Listeria monocytogenes on cold-smoked salmon. Int. J. Food Microbiol. 127, 235–240. doi: 10.1016/j.ijfoodmicro.2008.07.012
Yoshida, C. M. P., Maciel, V. B. V., Mendonça, M. E. D., and Franco, T. T. (2014). Chitosan biobased and intelligent films: monitoring pH variations. LWT- Food Sci. Technol. 55, 83–89. doi: 10.1016/j.lwt.2013.09.015
Yoshida, K., Mori, M., and Kondo, T. (2009). Blue flower color development by anthocyanins: from chemical structure to cell physiology. Nat. Prod. Rep. 26, 884–915. doi: 10.1039/b800165k
Yousefi, H., Su, H.-M., Imani, S. M., Alkhaldi, K., Filipe, C. D. M., and Didar, T. F. (2019). Intelligent food packaging: a review of smart sensing technologies for monitoring food quality. ACS Sens. 4, 808–821. doi: 10.1021/acssensors.9b00440
Zhai, X., Li, Z., Shi, J., Huang, X., Sun, Z., Zhang, D., et al. (2019). A colorimetric hydrogen sulfide sensor based on gellan gum-silver nanoparticles bionanocomposite for monitoring of meat spoilage in intelligent packaging. Food Chem. 290, 135–143. doi: 10.1016/j.foodchem.2019.03.138
Zhai, X., Li, Zhang, J., Shi, J., Zou, X., Huang, X., et al. (2018). Natural biomaterial-based edible and pH-sensitive films combined with electrochemical writing for intelligent food packaging. J. Agric. Food Chem. 66, 2836–12846. doi: 10.1021/acs.jafc.8b04932
Zhai, X., Shi, J., Zou, X., Wang, S., Jiang, C., Zhang, J., et al. (2017). Novel colorimetric films based on starch/polyvinyl alcohol incorporated with roselle anthocyanins for fish freshness monitoring. Food Hydrocolloid. 69, 308–317. doi: 10.1016/j.foodhyd.2017.02.014
Zhang, H., Hou, A., Xie, K., and Gao, A. (2019). Smart color-changing paper packaging sensors with pH sensitive chromophores based on azo-anthraquinone reactive dyes. Sens. Actuat. B 286, 362–369. doi: 10.1016/j.snb.2019.01.165
Keywords : bio-based, smart packaging, food packaging, food safety, bio-based sensor
Citation: Halonen N, Pálvölgyi PS, Bassani A, Fiorentini C, Nair R, Spigno G and Kordas K (2020) Bio-Based Smart Materials for Food Packaging and Sensors – A Review. Front. Mater. 7:82. doi: 10.3389/fmats.2020.00082
Received: 20 December 2019; Accepted: 20 March 2020; Published: 15 April 2020.
Reviewed by:
Copyright © 2020 Halonen, Pálvölgyi, Bassani, Fiorentini, Nair, Spigno and Kordas. This is an open-access article distributed under the terms of the Creative Commons Attribution License (CC BY) . The use, distribution or reproduction in other forums is permitted, provided the original author(s) and the copyright owner(s) are credited and that the original publication in this journal is cited, in accordance with accepted academic practice. No use, distribution or reproduction is permitted which does not comply with these terms.
*Correspondence: Niina Halonen, [email protected]
This article is part of the Research Topic
Materials for Sensing Applications

An official website of the United States government
The .gov means it’s official. Federal government websites often end in .gov or .mil. Before sharing sensitive information, make sure you’re on a federal government site.
The site is secure. The https:// ensures that you are connecting to the official website and that any information you provide is encrypted and transmitted securely.
- Publications
- Account settings
Preview improvements coming to the PMC website in October 2024. Learn More or Try it out now .
- Advanced Search
- Journal List
- v.13(3); 2022 May
Perspective: Soy-based Meat and Dairy Alternatives, Despite Classification as Ultra-processed Foods, Deliver High-quality Nutrition on Par with Unprocessed or Minimally Processed Animal-based Counterparts
Mark messina.
Soy Nutrition Institute Global, Washington, DC, USA
John L Sievenpiper
Departments of Nutritional Sciences and Medicine, Temerty Faculty of Medicine, University of Toronto, Toronto, Ontario, Canada
Division of Endocrinology and Metabolism, Department of Medicine, St. Michael's Hospital, Toronto, Ontario, Canada
Li Ka Shing Knowledge Institute, St. Michael's Hospital, Toronto, Ontario, Canada
Patricia Williamson
Scientific and Regulatory Affairs, Research and Development, Cargill, Wayzata, MN, USA
Jessica Kiel
Scientific and Clinical Affairs, Medifast, Inc., Baltimore, MD, USA
John W Erdman, Jr
Department of Food Science and Human Nutrition, Division of Nutritional Sciences and Beckman Institute, University of Illinois at Urbana/Champaign, Urbana, IL, USA
In many non-Asian countries, soy is consumed via soy-based meat and dairy alternatives, in addition to the traditional Asian soyfoods, such as tofu and miso. Meat alternatives are typically made using concentrated sources of soy protein, such as soy protein isolate (SPI) and soy protein concentrate (SPC). Therefore, these products are classified as ultra-processed foods (UPFs; group 4) according to NOVA, an increasingly widely used food-classification system that classifies all foods into 1 of 4 groups according to the processing they undergo. Furthermore, most soymilks, even those made from whole soybeans, are also classified as UPFs because of the addition of sugars and emulsifiers. Increasingly, recommendations are being made to restrict the consumption of UPFs because their intake is associated with a variety of adverse health outcomes. Critics of UPFs argue these foods are unhealthful for a wide assortment of reasons. Explanations for the proposed adverse effects of UPFs include their high energy density, high glycemic index (GI), hyper-palatability, and low satiety potential. Claims have also been made that UPFs are not sustainably produced. However, this perspective argues that none of the criticisms of UPFs apply to soy-based meat and dairy alternatives when compared with their animal-based counterparts, beef and cow milk, which are classified as unprocessed or minimally processed foods (group 1). Classifying soy-based meat and dairy alternatives as UPFs may hinder their public acceptance, which could detrimentally affect personal and planetary health. In conclusion, the NOVA classification system is simplistic and does not adequately evaluate the nutritional attributes of meat and dairy alternatives based on soy.
Statement of Significance : NOVA classifies soymilk and soy-based meat alternatives as ultra-processed foods (UPFs). However, criticisms of UPFs are not applicable to these foods when they are compared with their animal-based counterparts, which are classified as unprocessed or minimally processed foods. Admonitions against soymilk and soy-based meat alternatives based on their NOVA classification may dissuade consumers from consuming foods that offer health and environmental benefits.
Introduction
Over the past decade, plant-based meats and plant-based milks have markedly increased in popularity ( 1 ) because of their health and environmental attributes, and concerns over animal welfare ( 2 ). With regard to the environment, Goldstein et al. ( 3 ) concluded that plant-based beef substitutes could substantially reduce US greenhouse gas emissions, water consumption, and agricultural land occupation. Although plant-based patties made from different combinations of grains and beans have long been traditional vegetarian fare, the newest generation of plant-based meats is specifically designed to approximate the aesthetic qualities (primarily texture, flavor, and appearance) and nutritional attributes of specific types of meat in order to appeal to a broader range of consumers ( 4 ).
Despite their increased popularity, and potential environmental advantages, plant-based meat alternatives and plant-based milks have been criticized for being “highly processed.” In fact, according to the NOVA food-classification system, most plant-based meat alternatives ( 5 , 6 ) and plant-based milks ( 7 ) are classified as ultra-processed foods (UPFs; group 4) (for a detailed description, see Text Box 1 ) ( 5 ). This system categorizes all foods and food products into 4 groups according to the extent and purpose of the industrial processing they undergo ( 5 , 8 ). In contrast to plant-based meat alternatives and plant-based milks, their animal-based counterparts (beef and cow milk) are classified as unprocessed or minimally processed foods (group 1). UPFs are industrial food and drink formulations made of food-derived substances and additives, often containing little or no whole foods ( 9 ). In their recent editorial, Meyer and Taillie ( 10 ) noted with alarm the increase in and overall high intake of UPFs among US youth.
The NOVA food-classification system
• Group 1: Unprocessed/minimally processed
○ No added ingredients (fruit, vegetables, nuts,grains, meat, milk)
• Group 2: Processed culinary ingredients
○ Oils, fats, butter, vinegars, sugar, and salt, eatenwith group 1
• Group 3: Processed
○ Mix of groups 1 and 2 (chiefly for preservation)
○ Smoked and cured meats, cheeses, fresh bread,bacon, salted/sugared nuts, tinned fruit, beerand wine
• Group 4: Ultra-processed
○ Made with non-home ingredients
○ Chemicals, colorings, sweeteners, and preserva-tives
○ Industrial breads, cereals, sausage, dressings,snacks
○ High fat, sugar, and salt content is common
Classifying plant-based meat alternatives and plant-based milks as UPFs may slow their acceptance among consumers because, in most studies, UPFs are associated with an array of adverse health effects, including obesity, cardiovascular disease, and overall mortality ( 11 ). In fact, Wickramasinghe et al. ( 12 ) recently recommended restricting the marketing of plant-based meat and dairy substitutes because of their degree of processing. However, the American Society for Nutrition (ASN) maintains that “processed foods are nutritionally important to American diets because they contribute to food security, ensuring that sufficient food is available, and nutrition security, ensuring that food quality meets human nutrient needs” ( 13 ). The ASN also noted that food-processing techniques such as enrichment and fortification can add essential nutrients that might otherwise be in short supply and can alter food profiles to decrease components that may be overconsumed ( 13 ). Processing can also limit microbial contamination and reduce foodborne illness ( 14 ). In other words, processing can make foods more healthful.
The conflicting viewpoints on processed foods, and specifically plant-based meats and plant-based milks, present a confusing picture to consumers, especially health and environmentally conscious individuals who are concerned about animal welfare. This Perspective argues that maligning plant-based meats and plant-based milks because of the processing they undergo is nutritionally unjustified and counterproductive to achieving the health and environmental goals of the WHO, as well as those of other health authorities and organizations ( 15–18 ). Note that several authors have provided detailed overall critiques of the NOVA food-classification system ( 19–24 ). Therefore, the intent of this Perspective is not to critique the NOVA system in general. Nor is it to argue for reclassifying plant-based meat alternatives or plant-based milks. Rather, it is to show that, despite their classification as UPFs, these foods compare well with their animal-based counterparts, which are classified as unprocessed or minimally processed foods.
Although this Perspective discusses plant-based meat alternatives and plant-based milks in general, for 2 reasons, emphasis is placed on soymilk and soy-based meat substitutes. One, because of the large acreage devoted to growing soybeans, this legume has the greatest potential for meeting the caloric and protein needs of a growing global population. Approximately 350 million metric tons of soybeans are produced annually, and although most of that is used for animal feed (∼95%), its use is dictated by consumer demands ( 25 ).
Two, soy protein has traditionally been viewed by researchers as the reference plant protein, in part because of its high quality, and for this reason, is often compared with animal proteins, such as casein. Consequently, compared with other concentrated plant proteins, extensive clinical research has been conducted on concentrated sources of soy protein, which are the primary protein sources used in the manufacture of plant-based meat alternatives ( 26 ). For example, the ability of soy protein to lower blood cholesterol concentrations has been studied clinically for more than 50 y ( 27 ). Meta-analyses ( 28–35 ) published over the past nearly 20 y indicate a reduction in LDL cholesterol, ranging from 3.2% ( 35 ) to 6% ( 32 ). The impact of soy protein on muscle protein synthesis ( 36–38 ) and gains in muscle mass and strength ( 39 ) have also been widely studied. To this point, the results of a recent meta-analysis of longer-term studies (6–36 wk in duration) found that soy protein supplementation performed as well as whey and animal protein supplementation in individuals engaged in resistance exercise training ( 39 ).
Overview of Plant-based Meat Alternatives and Plant-based Milks
Role in meal planning.
Many authors have recommended a shift toward a plant-based diet ( 15 , 40–43 ), although the emphasis is typically on the consumption of whole foods or minimally processed foods, including whole grains, fruits, vegetables, nuts, legumes, and healthy oils ( 12 ). However, while these foods are nutritionally desirable, they are unlikely to fully address the orosensory preferences and practical needs of most consumers.
Legumes are an inexpensive, nutrient-rich source of protein ( 44 ), the consumption of which is recommended by health authorities throughout the world ( 45–48 ). Even so, legumes play a small role in the diets of developed countries and their intake is not expected to increase in the coming years in any region in the world ( 49 ). Furthermore, because pulses (grain legumes) are not an important part of Western diets, they require some education about how to cook and prepare them and how to incorporate them into recipes ( 50 ). As noted by van der Weele et al. ( 51 ), pulses are not novel from either a societal or technological point of view, and they have an unfavorable reputation as being old-fashioned.
In contrast to legumes, meat intake is expected to markedly increase over the next 30 y in many developing regions ( 52 , 53 ). Therefore, plant-based meat alternatives that imitate many of the properties of meat are more likely to impact consumption trends, and thus address environmental concerns, than is the direct consumption of legumes and beans. Research indicates that, while vegetarian and vegan consumers will accept plant-based meat alternatives that lack meat-like sensory properties, omnivorous and flexitarian consumers prefer alternatives that resemble animal-based protein as much as possible ( 54–57 ). In contrast, a recent UK survey found that most meat-eaters agree with the ethical and environmental arguments for vegetarianism/veganism but do not follow these diets because of practical reasons relating to taste, price, and convenience ( 58 ).
Detzel et al. ( 59 ) noted that, despite being highly processed, high-quality, plant-based, protein-rich foods can help reduce the environmental impact of food consumption while appealing to potential user groups beyond dedicated vegetarians and vegans. Furthermore, according to Lonkila and Kaljonen ( 60 ), consumers want convenient products that are easy to use and cook, attributes that are associated with meat and milk. Plant-based meat alternatives and plant-based milks are designed to meet these consumer preferences and can easily substitute for animal protein without requiring modification of meal patterns or food habits ( 61 , 62 ).
Also, because animal products, and especially meat, play an important role in structuring meals ( 62 , 63 ), plant-based substitutes that have the same functional properties allow an easy transition from animal-based to plant-based diets ( 64 ). Other alternative protein sources such as cultured meat, algae, and insects require more technological change than plant proteins, as well as requiring more social-institutional change for their acceptance ( 51 ). According to Hoek et al. ( 65 ), replacement of meat is most likely to be achieved by significantly improving the sensory quality of meat substitutes, but decreasing the cost and increasing the availability of these products are also important for greater consumer acceptance ( 66 ).
Finally, evidence suggests that the food environment is an important determinant of food consumption ( 67 , 68 ) and that certain eating context patterns, such as eating alone or eating while watching television, may promote the consumption of UPFs ( 69 , 70 ). Since plant-based meats and plant-based milks are designed to be used in the same way as their animal-based counterparts, the food environment does not favor 1 type (animal or plant) of milk or meat over the other.
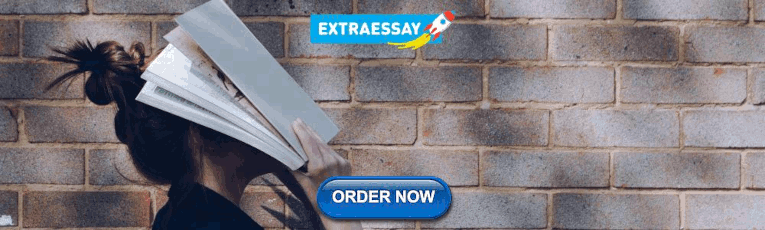
Nutritional implications
Recent research has addressed calls to gain a better understanding of the nutritional and health implications of plant-based substitutes, especially when replacing meat and dairy products ( 12 ). For example, Salomé et al. ( 61 ) assessed the effects of plant-based substitutes on the nutritional quality of the French diet by simulating separately the replacement of meat, milk, and dairy desserts with 96 plant-based substitutes. These authors found that overall plant-based substitutes had small and heterogeneous effects on diet quality and nutrient security, although plant-based substitutes that include legumes, such as soy, were shown to be more nutritionally adequate substitutes for animal products than other plant-based substitutes ( 61 ).
These overall findings align with the conclusion of Bohrer ( 71 ), that modern meat analogues can offer roughly the same composition of nutrients as traditional meat products. Similarly, Farsi et al. ( 72 ) concluded that plant-based meat alternatives can be a healthful replacement for meat, but also emphasized the need to choose options that are low in sodium and sugar, and high in fiber, protein, and nutrient density. From a protein perspective, these authors recommended choosing soy-based and mycoprotein-based (protein derived from fungi for human consumption) meat alternatives, but also noted the high sodium content of soy-based alternatives.
More in-depth analysis comes from van Vliet et al. ( 73 ), who found that, despite similarities based on front-of-package nutrition information, metabolomic profile abundances between a soy-based meat alternative (18 samples of the same product) and grass-fed ground beef (18 samples) differed by 90% (171 out of 190 profiled compounds; P < 0.05). However, the impact, if any, of these differences on the health status of the individuals consuming these products was not determined. Furthermore, all foods have vastly different metabolic profiles, including even those within the same botanical group ( 74 , 75 ).
Direct experimental insight about health outcomes comes from Crimarco et al. ( 76 ), who compared the effect on nutrient intake and cardiovascular disease (CVD) markers of consuming ∼2.5 servings/d of plant-based meat (pea- and soy protein-based) with meat-based counterparts over an 8-wk period. In response to the plant-based meats, concentrations of LDL cholesterol ( 77 ) and trimethylamine-N-oxide ( 78 ), a proposed but not established CVD risk factor ( 79 ), were statistically significantly reduced. In terms of nutrient intake, there were no differences in sodium or protein intake, whereas in response to the consumption of plant-based products, saturated fat was lower and fiber intake higher, although the fiber difference was not statistically significant. More recently, the replacement of ∼5 servings/wk of meat with plant-based meat alternatives led to favorable changes (e.g., an increase in butyrate-metabolizing potential and a decrease in the Tenericutes phylum) in the gut microbiome over a 4-wk period ( 80 ).
Soy protein quality
Until recently, most of the research aimed at determining the quality of soy protein focused on the soy protein ingredients rather than traditional Asian soyfoods. The soy protein ingredients, soy protein isolate (SPI), soy protein concentrate (SPC), and soy flour, are composed of ≥90%, 65–90%, and 50–65% protein, respectively ( 26 ). An advantage of these concentrated sources of soy protein is that they more easily allow greater amounts of protein to be incorporated into experimental diets, especially into products such as beverages or baked goods (e.g., muffins) that can be made indistinguishable from products containing the control protein. This enables better participant blinding and enhanced compliance.
The high quality of soy protein was firmly established by a series of nitrogen balance studies by Young and colleagues conducted in the early 1980s ( 81–86 ). In the early 1990s, the protein digestibility corrected amino acid score (PDCAAS) was adopted by the US FDA and FAO as the method of choice for determining protein quality. Utilizing 2 different laboratories, Hughes et al. ( 87 ) determined that the untruncated PDCAAS of 3 different SPIs ranged from 0.95 to 1.02 and the scores for the single SPC were 1.02 and 1.05. These values are similar to those determined by Rutherfurd et al. ( 88 ) for SPI and by Mathai et al. ( 89 ) for SPI and soy flour. According to the USDA, to qualify as a high-quality protein requires a score of at least 0.8.
In 2011, an FAO consultation recommended transitioning from the PDCAAS to the digestible indispensable amino acid score (DIAAS) ( 90 ). Given that some methodological issues remain to be resolved ( 91 ), it will likely be several years before the DIAAS is accepted by regulatory bodies. Preliminary data using the DIAAS also support the high quality of soy protein ( 88 , 89 ), although, in general, the quality of plant protein is rated slightly lower using this method compared with the PDCAAS ( 88 ). Very recently, Fanelli et al. ( 92 ) determined that the DIAAS for the Impossible Burger [(Impossible Foods) primary protein source is soy] was similar to the DIAAS for 80% ground beef when calculated using the indispensable amino acid (IAA) pattern for the older child, adolescent, and adult.
Applicability of criticisms of processed foods to soy-based meats and soymilk
As previously noted, the consumption of UPFs has been associated with a range of adverse health outcomes ( 11 ). Diets high in UPFs are associated with poor diet quality ( 93 ), but there is debate about the extent to which diet quality accounts for the associations between UPF intake and adverse health outcomes ( 19 , 94 ). Many of the effects of processing will be identified by existing food-classification systems (nutritional rating systems) that are based exclusively on nutrient (and fiber) content. This is true for several of the major criticisms of UPFs, such as their high energy density ( 95 , 96 ), high glycemic index (GI) ( 97 ) or high glycemic glucose equivalent ( 98 ), hyper-palatability ( 95 ), and low satiety potential ( 97 ). However, as noted by others, processing can lead to textural and structural changes to the food matrix not identified by nutritional rating systems that can speed up the rate at which UPFs are consumed ( 96 , 99 , 100 ). Reducing the orosensory exposure time of a food can delay the onset of satiation ( 101 ). UPFs have been shown to be less satiating than minimally processed foods ( 97 , 102 ), which can promote increased energy intake ( 103 ).
Energy intake rate may be an especially important contributor to the links between UPF intake and obesity. Forde et al. ( 100 ) recently showed, after pooling data from 5 studies that measured energy intake rates across a total sample of 327 foods, that when going from unprocessed, to processed, to ultra-processed, the average energy intake rate increased from 35.5 ± 4.4 to 53.7 ± 4.3 to 69.4 ± 3.1 kcal/min ( P < 0.05), respectively. Additional explanations for the harmful effects of UPFs include the presence of artificial food additives ( 104–106 ) and artificial sweeteners, which have been linked to alterations to the gut microbiota ( 106–108 ), although not reliably in humans ( 109 , 110 ). Also, food processing, and particularly heat treatment, may produce contaminants (e.g., acrylamide) in UPFs that may increase cancer risk ( 111 ). Bisphenol A, a contaminant suspected of migrating from plastic packaging of UPFs, has been shown to possess endocrine-disruptive properties ( 112 ).
Finally, although not related to personal health, claims have also been made that UPFs are not sustainably produced ( 9 , 113 ), which is likely to become an increasingly important consideration in the formulation of dietary guidelines. According to the Society for Nutrition Education “environmental sustainability should be inherent in dietary guidance, whether working with individuals or groups about their dietary choices or in setting national dietary guidance” ( 114 ).
There are a variety of soy-based meat alternatives and soymilks on the market. For the examination of the applicability of the criticisms of UPFs to soy-based meat alternatives and soymilk, 5 soy protein–based burgers were compared with 80% lean beef ( Table 1 ) and 2 soymilks were compared with whole and 2% cow milk, the 2 most commonly consumed milks in the United States ( Table 2 ). Silk Original Soymilk and Silk Organic Unsweetened Soymilk were chosen for comparison because these products are the top 2 selling stock-keeping units in the US refrigerated soy plant-based beverage category. Silk is the leading brand based on US national sales data (Kristie Leigh, Danone North American, personal communication September 10, 2021).
Nutrient, caloric, and fiber content of lean beef and selected soy-based burgers 1
Nutrient, caloric, and fiber content of cow milk and soy milk 1
Energy density
The connection between energy density, UPF intake, and weight gain was highlighted by a recent 2-wk crossover study involving 20 overweight adults ( 96 ). When consuming the diet composed primarily of UPFs, participants gained weight, whereas weight was lost during the unprocessed diet phase. The much higher nonbeverage energy density (2.147 vs. 1.151 kcal/g) of the UPF diet was suggested as being a key factor contributing to the weight gain. The energy density (kilocalories/gram) of the soy burgers in Table 1 is similar to or lower than that of beef. On a percentage calorie basis, the soy-based burgers contain similar or higher amounts of protein, but similar or lower amounts of fat and, unlike the beef, contain dietary fiber. It is reasonable to speculate that the fiber content of soy-based burgers could promote satiety relative to beef ( 115 ). Therefore, there is little reason to suggest the eating rate (grams/minute) or, more importantly, the energy intake rate (kilocalories/minute) of the soy burgers would be greater than beef. The soy-based burgers do contain carbohydrate, although much of that is fiber. As somewhat of an aside, although only one of the soy-based burgers qualifies as a high-sodium food (≥460 mg/serving), 2 others come close to doing so. Therefore, manufacturers of soy-based meat alternatives should be encouraged to keep sodium content in mind when producing new, or reformulating, products.
Table 2 shows that the soymilks have a lower energy density than both whole and 2% cow milk and contain similar amounts of protein. The major difference between milk types is with respect to carbohydrate content: the soymilks contain fiber (2 g/serving) and sucrose, whereas cow milk has no fiber and contains lactose. However, the soymilks contain a lower percentage of calories from carbohydrate and are lower in sugar. Neither the energy density nor macronutrient content suggests that soymilk would result in a faster eating rate or greater energy intake rate than cow milk. Although not necessarily related to satiety, it is notable from an overall health perspective that, as a percentage of calories, the soymilks and soy burgers are lower in saturated fat than their animal-based counterparts.
Glycemic response
There is convincing evidence that reducing postprandial glycemia is a desirable physiological goal ( 116 , 117 ) and that doing so reduces the risk of developing diabetes ( 118 , 119 ) and coronary artery disease ( 120 ). As noted, the impact of processing on the GI has been highlighted as a factor possibly contributing to the adverse health outcomes associated with UPF intake ( 97 ). Processing can affect the GI of foods ( 121–123 ) even independently of fiber content ( 124 ).
The American Diabetes Association recommends consumption of low (<55) and medium ( 56–69 ) GI foods for people with diabetes and other individuals looking to control blood sugar concentrations. Both soymilk and cow milk are acceptable foods according to these criteria ( 125 ). The GI and the glycemic load (GL; a measure that combines the GI with the amount of carbohydrate in a food) of soymilks depend upon the amount of added sugar ( 126 ).
Serrano et al. ( 127 ) concluded that soymilk was a low-GI food based on the results of a crossover study in which 29 young adults ingested 500 mL water, 500 mL glucose solution (20.5 g/500 mL), or 500 mL of soymilk on 3 separate occasions. Sun et al. ( 128 ) found that, in Chinese participants, coingestion of cow milk or soymilk with bread lowered the postprandial blood glucose response relative to bread alone. Also, Law et al. ( 129 ) found no difference between the effect of cow milk and soymilk on blood glucose or insulin concentrations at 180 min after consuming a meal that, in addition to each milk, contained bread and jam (cow milk was 2% fat and the soymilk was made using SPI). Finally, Atkinson et al. ( 121 ) reported that the GIs of cow milk (full-fat) and soymilk were 39 and 34, respectively, although more recent work from this group reported an average GI of only 25 for 13 different cow milks of variable fat content ( 130 ). The evidence overall suggests that there is nothing inherent to soymilk that would cause it to have a higher GI or GL than cow milk.
Hyper-palatability/satiety
Preliminary research indicates that many UPFs that are often high in fat and have a high GL are hyper-palatable and linked to addictive-like eating behaviors ( 131 , 132 ). However, recent research shows that UPFs are not in and of themselves hyper-palatable ( 133 ). Furthermore, and more importantly, research shows that soymilk is not viewed as hyper-palatable in comparison to cow milk ( 134–138 ). With regard to meat, from a sensory perspective, it is the gold standard that the new generation of plant-based meat alternatives is trying to emulate (as opposed to a black bean burger, which is not designed to mimic the taste of meat) ( 4 ). While this standard may be matched, it is not clear how it could be exceeded, a conclusion that aligns with recent survey results ( 139 ).
As noted previously, one concern about UPFs is that their physical and structural characteristics may result in lower satiety potential and higher glycemic response ( 97 ) and may, because of their higher energy density, be consumed at a faster energy intake rate than less-processed foods ( 96 ). These attributes could lead to an increased energy intake, which, in turn, could lead to obesity and associated adverse health outcomes. However, evidence indicates that these concerns do not apply to soy-based meats or soymilk.
No clinical studies were identified that compared the effects of a soy-based burgers with meat, or soymilk with cow milk, on weight loss. However, in the Study With Appetizing Plantfood-Meat Eating Alternative Trial (SWAP-MEAT), weight loss occurred in the group consuming plant-based meat alternatives, some of which were based on pea protein and some on soy protein ( 76 ). Therefore, at the very least, the results indicate that plant-based meats are not inherently obesogenic. Also, meal replacements containing isolated proteins led to greater weight loss than traditional weight-loss diets ( 140–142 ), which suggests that, at the least, concentrated sources of proteins such as SPI and SPC do not promote weight gain.
Two studies compared beef and products made with soy protein ingredients on metabolic parameters related to weight loss. In one, obese participants consumed either a vegetarian (soy) high-protein, weight-loss (HPWL) diet or a meat-based HPWL for 2 wk and then crossed over to the opposite diet ( 143 ). Assessments of appetite control, weight loss, and gut hormone profile (glucagon like peptide 1, ghrelin, and peptide YY) did not differ between the diets. The soy-HPWL and meat-HPWL diets were each composed of 30% protein, 30% fat, and 40% carbohydrate. The meat-HPWL diet was based on chicken and beef; the soy-HPWL diet was based on soy protein ingredients. In the other study, meals (400 kcal) containing beef or SPC were matched for macronutrients and fiber or serving size (2 different arms) and consumed by 21 young, healthy adults ( 144 ). The type of protein consumed within a mixed meal had little effect on appetite, satiety, or food intake.
Finally, a study in 96 healthy adults found no difference between the mean (±SD) chewing time associated with 5 g chicken (16.9 ± 5.6 s) and 5 g vegetarian (soy-based) chicken (17.9 ± 6.2 s), although the former resulted in a bolus of chicken that had significantly more ( P < 0.001) and smaller ( P < 0.001) particles than vegetarian chicken ( 145 ). The similar chewing time suggests that energy intake rate is not likely to differ between meat and soy-based meat alternatives.
Sustainability
As noted earlier, claims have been made that UPFs are not sustainably produced ( 9 , 113 ), which is likely to become an increasingly important consideration in the formulation of dietary guidelines ( 114 ). As discussed below, evidence indicates that soy-based meat and dairy products have environmental advantages. However, it is important to acknowledge that, as is the case for the impact of diet on health, there are widely differing opinions about the effects of diet on climate and its potential to affect global warming ( 146 , 147 ). Establishing the global warming potential (GWP) of a dietary pattern or food is a complex process that involves a scientific understanding that continues to evolve. The environmental impact of any food, whether it be soymilk or soy-based meat, will depend, in part, upon the specific composition of the product in question.
Legumes have been shown to have an extremely low GWP, in comparison to nearly all other protein sources ( 148–151 ), although this depends in part upon the management of the agro-ecosystem used (e.g., mono-cropping vs. conservation agriculture) ( 152 ). In 2011, González et al. ( 153 ) determined that, of the 22 plant and animal protein sources evaluated, soybeans were the most efficiently produced and provided the most protein (grams) per greenhouse gas emissions [GHGE; kilogram carbon dioxide (kg CO 2 ) equivalents]. Tessari et al. ( 154 ) emphasized that, when considering the environmental impact of foods, it is important to consider nutritional value and, in particular, IAA content. When this metric was used, there was little difference between animal and plant protein sources, except for soybeans, which exhibited the smallest environmental footprint.
Soybeans, like all legumes, can fix nitrogen because of the bacterial symbionts (rhizobia) that inhabit nodules on their roots. The amount of ammonia produced by rhizobial fixation of nitrogen by legumes rivals that of the world's entire fertilizer industry ( 155 ). The fact that legumes do not require nitrogen fertilizer for growth represents an important environmental advantage because half the nitrogen applied to fields for crop fertilization is thought to be lost into the environment, creating environmental concerns due to entry in surface and groundwater ( 156 , 157 ).
While the environmental impact of soybean production is an important consideration, it is only 1 factor affecting the environmental impact of soy protein ingredients and the products made using them. Therefore, the conclusion by van Mierlo et al. ( 158 ) that soy protein ingredients are keys to mimicking the nutrient profile of meat, while minimizing environmental impact with regard to climate change, land use, water use, and fossil fuel depletion, is notable. This conclusion agrees with work by Thrane et al. ( 159 ). Reducing water and land use is particularly notable. Several groups have determined that the GWP of meat alternatives is lower than that of meat ( 3 , 160–164 ). For example, the GWP of an Impossible Burger was determined to be lower than that of a beef burger and to require less land and water for its production ( 165 ).
With respect to soymilk, research has shown that its production requires considerably less water than to produce cow milk ( 166 , 167 ). Also, shelf-stable soymilk was found to produce far fewer GHGE than shelf-stable cow milk ( 168 ). In agreement, Poore and Nemecek ( 148 ) found that, for each of the 5 criteria considered (GHGE, land use, acidification, eutrophication, water scarcity), and when expressed on a per-protein basis, soymilk production always resulted in a lower environmental impact than cow milk. Very recently, Coluccia et al. ( 169 ) also concluded that soymilk has a lower carbon footprint than cow milk.
Summary and Conclusions
The increased role of plant-based meat alternatives and plant-based milks in the diets of consumers around the world necessitates that scientists and health professionals have a detailed understanding of their nutritional, health, and environmental attributes, and considerable progress in this regard has been made. Nevertheless, plant-based products have been criticized for being overly processed ( 12 ). While it is undoubtedly true that many UPFs are not nutrient dense ( 170 , 171 ), it is important not to assume that “ultra-processed” equals poor nutritional quality, since quality does not depend solely on the intensity or complexity of processing but on the final composition of the food itself ( 172 ).
As discussed, soy-based meats and soymilk compare favorably with their animal-based counterparts nutritionally. Further, there is no evidence that the major criticisms of UPFs [including high energy density ( 95 , 96 ), high GI ( 97 ), hyper-palatability ( 95 ), and low satiety potential ( 97 )] apply to these soy-based products. Certainly, within each category of plant-based meat alternatives and plant-based milks there will be variations in nutrient content because of differences in the protein source, fat source, and the extent of fortification. Therefore, consumers will need to compare Nutrition Facts panels. Consumers are best advised to choose soymilks that are protein-rich (6–8 g/cup), low in sugar, and that are fortified with calcium and vitamin D, and to keep sodium content in mind when choosing plant-based meats. However, admonitions against the consumption of products simply because they are classified as UPFs are unwarranted and may impair society's acceptance of plant-based diets—thus preventing the related health and environmental advantages from being realized.
While it may be true that the consumption of many UPFs should be discouraged based on nutrient content, this generalization does not apply to all such foods. Rather, the nutritional composition of the final product and its impact on health and sustainability should serve as the ultimate guide concerning the merits of a specific food, not the extent to which that food is considered processed. In summary, in the case of soy-based meat alternatives and soymilks, the NOVA classification system is overly simplistic and of little utility for evaluating the true nutritional attributes of these foods.
Acknowledgments
The authors’ responsibilities were as follows—MM: wrote the initial draft of the manuscript with contributions from JWE and JLS; and all authors: reviewed and commented on subsequent drafts of the manuscript and read and approved the final manuscript.
Author disclosures: MM is employed by the Soy Nutrition Institute Global, an organization that receives funding from the United Soybean Board and industry members who are involved in the manufacture and/or sale of soyfoods and/or soybean components. JLS has received research support from the Canadian Foundation for Innovation, Ontario Research Fund, Province of Ontario Ministry of Research and Innovation and Science, Canadian Institutes of health Research (CIHR), Diabetes Canada, PSI Foundation, Banting and Best Diabetes Centre (BBDC), American Society for Nutrition (ASN), INC International Nut and Dried Fruit Council Foundation, National Dried Fruit Trade Association, National Honey Board (the USDA honey “Checkoff” program), International Life Sciences Institute (ILSI), Pulse Canada, Quaker Oats Center of Excellence, The United Soybean Board (the USDA soy “Checkoff” program), The Tate and Lyle Nutritional Research Fund at the University of Toronto, The Glycemic Control and Cardiovascular Disease in Type 2 Diabetes Fund at the University of Toronto (a fund established by the Alberta Pulse Growers), and The Nutrition Trialists Fund at the University of Toronto (a fund established by an inaugural donation from the Calorie Control Council). He has received food donations to support randomized controlled trials from the Almond Board of California, California Walnut Commission, Peanut Institute, Barilla, Unilever/Upfield, Unico/Primo, Loblaw Companies, Quaker, Kellogg Canada, WhiteWave Foods/Danone, Nutrartis, and Dairy Farmers of Canada. He has received travel support, speaker fees, and/or honoraria from Diabetes Canada, Dairy Farmers of Canada, FoodMinds LLC, International Sweeteners Association, Nestlé, Pulse Canada, Canadian Society for Endocrinology and Metabolism (CSEM), GI Foundation, Abbott, General Mills, Biofortis, ASN, Northern Ontario School of Medicine, INC Nutrition Research and Education Foundation, European Food Safety Authority (EFSA), Comité Européen des Fabricants de Sucre (CEFS), Nutrition Communications, International Food Information Council (IFIC), Calorie Control Council, International Glutamate Technical Committee, and Physicians Committee for Responsible Medicine. He has or has had ad hoc consulting arrangements with Perkins Coie LLP, Tate & Lyle, Wirtschaftliche Vereinigung Zucker eV, Danone, and Inquis Clinical Research. He is a member of the European Fruit Juice Association Scientific Expert Panel and former member of the Soy Nutrition Institute (SNI) Scientific Advisory Committee. He is on the Clinical Practice Guidelines Expert Committees of Diabetes Canada, European Association for the study of Diabetes (EASD), Canadian Cardiovascular Society (CCS), and Obesity Canada/Canadian Association of Bariatric Physicians and Surgeons. He serves or has served as an unpaid scientific advisor for the Food, Nutrition, and Safety Program (FNSP) and the Technical Committee on Carbohydrates of ILSI North America. He is a member of the International Carbohydrate Quality Consortium (ICQC), Executive Board Member of the Diabetes and Nutrition Study Group (DNSG) of the EASD, and Director of the Toronto 3D Knowledge Synthesis and Clinical Trials foundation. His wife is an employee of AB InBev. PW is employed by Cargill, Inc, a global food company headquartered in Wayzata, MN. Cargill produces soy-based food and industrial products. JK is employed by Medifast Inc., a nutrition and weight-management company based in Baltimore, Maryland, that uses soy protein in many of its products. JWE is a scientific advisory to the Soy Nutrition Institute Global.
Perspective articles allow authors to take a position on a topic of current major importance or controversy in the field of nutrition. As such, these articles could include statements based on author opinions or point of view. Opinions expressed in Perspective articles are those of the author and are not attributable to the funder(s) or the sponsor(s) or the publisher, Editor, or Editorial Board of Advances in Nutrition . Individuals with different positions on the topic of a Perspective are invited to submit their comments in the form of a Perspectives article or in a Letter to the Editor.
Abbreviations used: CVD, cardiovascular disease; DIAAS, digestible indispensable amino acid score; GHGE, greenhouse gas emissions; GI, glycemic index; GL, glycemic load; GWP, global warming potential; HPWL, high-protein, weight-loss; IAA, indispensable amino acid; PDCAAS, protein digestibility corrected amino acid score; SPC, soy protein concentrate; SPI, soy protein isolate; UPF, ultra-processed food.
Contributor Information
Mark Messina, Soy Nutrition Institute Global, Washington, DC, USA.
John L Sievenpiper, Departments of Nutritional Sciences and Medicine, Temerty Faculty of Medicine, University of Toronto, Toronto, Ontario, Canada. Division of Endocrinology and Metabolism, Department of Medicine, St. Michael's Hospital, Toronto, Ontario, Canada. Li Ka Shing Knowledge Institute, St. Michael's Hospital, Toronto, Ontario, Canada.
Patricia Williamson, Scientific and Regulatory Affairs, Research and Development, Cargill, Wayzata, MN, USA.
Jessica Kiel, Scientific and Clinical Affairs, Medifast, Inc., Baltimore, MD, USA.
John W Erdman, Jr, Department of Food Science and Human Nutrition, Division of Nutritional Sciences and Beckman Institute, University of Illinois at Urbana/Champaign, Urbana, IL, USA.
Biobased Nanomaterials in Nutraceuticals
- First Online: 14 May 2024
Cite this chapter
- Joyeta Ghosh ORCID: orcid.org/0000-0001-9619-1793 2 ,
- Sudrita Roy Choudhury ORCID: orcid.org/0000-0002-5205-0590 3 ,
- Khushboo Singh ORCID: orcid.org/0000-0001-6374-0485 3 ,
- Madan Mohan Gupta ORCID: orcid.org/0000-0002-5224-4774 4 &
- Deepak Sharma 5
Research has yet to demonstrate the biobased materials’ significant contribution as a renewable, limitless source of natural constituents required for biomedical and pharmaceutical applications (including forestry residues, such as tree branches, bark, or residues of industrial crops and bushes). On the other hand, a wide application of nanotechnology is expanding in food science and food industry disciplines as the fastest-growing as well as the most promising nanomaterial applications. As a result, the use of biocompatible nanomaterials is growing in significance and is gradually turning into a necessity for human exposure, particularly in the context of food consumption. Actually, the use of high-performance, lightweight, novel, and environmentally friendly components in place of traditional or conventional nonbiodegradable materials is becoming more and more possible thanks to bio-nanocomposite materials. Developing biobased nanotechnology has demonstrated considerable potential for use in functional foods and nutraceuticals to enhance human health. Currently, some of the most important biopolymers and extractives, such as sustainable feedstock, are cellulose, hemicellulose, lignin, and chitosan. These materials are used to make high-value-added products. These also comprise biopharmaceuticals, biobased materials, pharmaceuticals, chemicals, and functional materials. In the context of the application of nutraceuticals or functional foods, maintaining their bioavailability in the human gut as well as maintaining prolonged functionality is one change the food industry is facing. In some situations, these bioactive substances may give the finished product an unfavorable organoleptic trait, which reduces consumer approval. In order to meet the expectations of consumers, efficient bioactives and biobased nanomaterials play a crucial role in such delivery systems. In order to profit from the health-promoting properties of bioactive components and to continue the market expansion for functional foods, scientific understanding regarding the development of effective bioactive component delivery into contemporary and diverse food products is becoming crucial. The present chapter is a review of biobased nanomaterials in nutraceuticals. It thoroughly reviewed the current status of research on nutraceuticals, functional foods, and foods for medical purposes. It also covered the most important pertinent guidelines, laws, and directives, as well as how these subjects specifically affect certain foods and beverages. The use of biobased nanomaterials helps in resolving environmental hazards due to the biodegradability and nontoxicity of several biopolymers in the nutraceutical-related food industry. Therefore, more in-depth research is necessary to address critical safety challenges as well as provide details on the dangers and risks associated with nanomaterials used in the food industry.
This is a preview of subscription content, log in via an institution to check access.
Access this chapter
- Available as PDF
- Read on any device
- Instant download
- Own it forever
- Available as EPUB and PDF
- Durable hardcover edition
- Dispatched in 3 to 5 business days
- Free shipping worldwide - see info
Tax calculation will be finalised at checkout
Purchases are for personal use only
Institutional subscriptions
Aadinath W, Bhushani A, Anandharamakrishnan C (2016) Synergistic radical scavenging potency of curcumin-in-β-cyclodextrin-in-nanomagnetoliposomes. Mater Sci Eng C 64:293–302. https://doi.org/10.1016/j.msec.2016.03.095
Article CAS Google Scholar
Aalinkeel R, Kutscher HL, Singh A, Cwiklinski K, Khechen N, Schwartz SA, Prasad PN, Mahajan SD (2018) Neuroprotective effects of a biodegradable poly(lactic-co-glycolic acid)-ginsenoside Rg3 nanoformulation: a potential nanotherapy for Alzheimer’s disease? J Drug Target 26:182–193
Article CAS PubMed Google Scholar
Abedini M, Shariatmadari F, Torshizi MAK, Ahmadi H (2018) Effects of zinc oxide nanoparticles on the egg quality, immune response, zinc retention, and blood parameters of laying hens in the late phase of production. J Anim Physiol Anim Nutr (Berl) 102:736–745
Acevedo-Fani A, Soliva-Fortuny R, Martín-Belloso O (2018) Photo-protection and controlled release of folic acid using edible alginate/chitosan nanolaminates. J Food Eng 229:72–82. https://doi.org/10.1016/j.jfoodeng.2017.03.024
Adetunji CO, Ogundolie FA, Ajiboye MD, et al. (2022) Nano-engineered sensors for food processing. Bio- and Nano-sensing Technologies for Food Processing and Packaging, pp. 151–166. https://doi.org/10.1039/9781839167966-00151
Aditya NP, Espinosa YG, Norton IT (2017) Encapsulation systems for the delivery of hydrophilic nutraceuticals: food application. Biotechnol Adv 35:450–457. https://doi.org/10.1016/j.biotechadv.2017.03.012
Ahmad M, Gani A (2021) Development of novel functional snacks containing nano-encapsulated resveratrol with anti-diabetic, antiobesity and antioxidant properties. Food Chem 352:129323. https://doi.org/10.1016/j.foodchem.2021.129323
Ahmadi M, Ahmadian A, Seidavi AR (2018) Effect of different levels of nano-selenium on performance, blood parameters, immunity and carcass characteristics of broiler chickens. Poult Sci J 6:99–108
Google Scholar
Alaarg A, Jordan NY, Verhoef JJF, Metselaar JM, Storm G, Kok RJ (2016) Docosahexaenoic acid liposomes for targeting chronic inflammatory diseases and cancer: an in vitro assessment. Int J Nanomedicine 11:5027–5040
Article CAS PubMed PubMed Central Google Scholar
Alarcón-Alarcón C, Inostroza-Riquelme M, Torres-Gallegos C et al (2018) Protection of astaxanthin from photodegradation by its inclusion in hierarchically assembled nano and microstructures with potential as food. Food Hydrocoll 83:36–44. https://doi.org/10.1016/j.foodhyd.2018.04.033
Alishahi A, Mirvaghefi A, Tehrani MR, Farahmand H, Koshio S, Dorkoosh FA, Elsabee MZ (2011) Chitosan nanoparticle to carry vitamin C through the gastrointestinal tract and induce the non-specific immunity system of rainbow trout (Oncorhynchus mykiss). Carbohydr Polym 86:142–146
Amalraj A, Gopi S, Thomas S, Haponiuk JT (2018) Cellulose nanomaterials in biomedical, food, and nutraceutical applications: a review. Macromol Symp 380. https://doi.org/10.1002/masy.201800115
Ameta SK, Rai AK, Hiran D, Ameta R, Ameta SC (2020) Use of nanomaterials in food science. Biog Nano Particl Use Agro Ecosyst 457:24. https://doi.org/10.1007/978-981-15-2985-6_24
Article Google Scholar
Anjugam M, Vaseeharan B, Iswarya A, Gobi N, Divya M, Thangaraj MP, Elumalai P (2018) Effect of β-1, 3 glucan binding protein based zinc oxide nanoparticles supplemented diet on immune response and disease resistance in Oreochromis mossambicus against Aeromonas hydrophila. Fish Shellfish Immunol 76:247–259
Anu Bhushani J, Anandharamakrishnan C (2014) Electrospinning and electrospraying techniques: potential food based applications. Trends Food Sci Technol 38:21–33. https://doi.org/10.1016/j.tifs.2014.03.004
Arora D, Jaglan S (2016) Nanocarriers based delivery of nutraceuticals for cancer prevention and treatment: a review of recent research developments. Trends Food Sci Technol 54:114–126. https://doi.org/10.1016/j.tifs.2016.06.003
Arzeni C, Pérez OE, LeBlanc JG, Pilosof AMR (2015) Egg albumin–folic acid nanocomplexes: performance as a functional ingredient and biological activity. J Funct Foods 18:379–386. https://doi.org/10.1016/j.jff.2015.07.018
Atia A, Gomaa A, Fliss I, Beyssac E, Garrait G, Subirade M (2016) A prebiotic matrix for encapsulation of probiotics: physicochemical and microbiological study. J Microencapsul 33:89–101
Azzi J, Jraij A, Auezova L et al (2018) Novel findings for quercetin encapsulation and preservation with cyclodextrins, liposomes, and drug-in-cyclodextrin-in-liposomes. Food Hydrocoll 81:328–340. https://doi.org/10.1016/j.foodhyd.2018.03.006
Babazadeh A, Ghanbarzadeh B, Hamishehkar H (2017) Phosphatidylcholine-Rutin complex as a potential nanocarrier for food applications. J Funct Foods 33:134–141. https://doi.org/10.1016/j.jff.2017.03.038
Bagherpour S, Alizadeh A, Ghanbarzadeh S, Mohammadi M, Hamishehkar H (2017) Preparation and characterization of Betasitosterol-loaded nanostructured lipid carriers for butter enrichment. Food Biosci 20:51–55
Bai DP, Lin XY, Huang YF, Zhang XF (2018) Theranostics aspects of various nanoparticles in veterinary medicine. Int J Mol Sci 19:3299
Article PubMed PubMed Central Google Scholar
Bhatt PC, Pathak S, Kumar V, Panda BP (2018) Attenuation of neurobehavioral and neurochemical abnormalities in animal model of cognitive deficits of Alzheimer’s disease by fermented soybean nanonutraceutical. Inflammopharmacology 26:105–118
Article PubMed Google Scholar
Bhushan B (2010) Introduction to nanotechnology. Springer Handb Nanotechnol 9:1–13. https://doi.org/10.1007/978-3-642-02525-9
Bioinicia (2016) Electrospinning and electrospraying highlights at NANOTEC 2016. In: Bioinicia. https://bioinicia.com/electrospinning-electrospraying-highlights-at-nanotec-2016/ . Accessed 4 Oct 2023
Bleeker EAJ, de Jong WH, Geertsma RE et al (2013) Considerations on the EU definition of a nanomaterial: science to support policy making. Regul Toxicol Pharmacol 65:119–125. https://doi.org/10.1016/j.yrtph.2012.11.007
Bochicchio S, Barba AA, Grassi G, Lamberti G (2016) Vitamin delivery: carriers based on nanoliposomes produced via ultrasonic irradiation. LWT Food Sci Technol 69:9–16. https://doi.org/10.1016/j.lwt.2016.01.025
Boholm Å, Larsson S (2019) What is the problem? A literature review on challenges facing the communication of nanotechnology to the public. J Nanopart Res 21:1–21. https://doi.org/10.1007/s11051-019-4524-
Boostani A, Sadeghi AA, Mousavi SN, Chamani M, Kashan N (2015) The effects of organic, inorganic, and nano-selenium on blood attributes in broiler chickens exposed to oxidative stress. Acta Sci Vet 43:1264
Bouwmeester H, Brandhoff P, Marvin HJP, Weigel S, Peters RJB (2014) State of the safety assessment and current use of nanomaterials in food and food production. Trends Food Sci Technol 40:200–210. https://doi.org/10.1016/j.tifs.2014.08.009
Braithwaite MC, Choonara YE, Kumar P et al (2015) A novel bile salts–lipase polymeric film-infused minitablet system for enhanced oral delivery of cholecalciferol. Pharm Dev Technol 21:832–846. https://doi.org/10.3109/10837450.2015.1069329
Brito-Oliveira TC, Molina CV, Netto FM, Pinho SC (2017) Encapsulation of beta-carotene in lipid microparticles stabilized with hydrolyzed soy protein isolate: production parameters, alpha-tocopherol coencapsulation and stability under stress conditions. J Food Sci 82:659–669. https://doi.org/10.1111/1750-3841.13642
Brown J (2022) Invited perspective: sanitation innovation holds promise but must consider risks to users. Environ Health Perspect 130:11301. https://doi.org/10.1289/ehp10609
Cai SJ, Wu CX, Gong LM, Song T, Wu H, Zhang LY (2012) Effects of nano-selenium on performance, meat quality, immune function, oxidation resistance, and tissue selenium content in broilers. Poultry Sci 91:2532–2539
Cai XX, Zhao LN, Wang SY, Rao PF (2015) Fabrication and characterization of the nano-composite of whey protein hydrolysate chelated with calcium. Food Funct 6:816–823
Carbone M, Donia DT, Sabbatella G, Antiochia R (2016) Silver nanoparticles in polymeric matrices for fresh food packaging. J King Saud Univ Sci 28:273–279. https://doi.org/10.1016/j.jksus.2016.05.004
Center for Veterinary Medicine Nanotechnology Programs (2018). https://www.fda.gov/ScienceResearch/SpecialTopics/Nanotechnology/ucm309682.htm . Accessed 1 Dec 2018
Chai J, Jiang P, Wang P et al (2018) The intelligent delivery systems for bioactive compounds in foods: physicochemical and physiological conditions, absorption mechanisms, obstacles and responsive strategies. Trends Food Sci Technol 78:144–154. https://doi.org/10.1016/j.tifs.2018.06.003
Chakraborty AP (2016) Chicken eggshell as calcium supplement tablet. Int J Sci Eng Manag 1:45–49
Chan TS, Galati G, Pannala AS et al (2003) Simultaneous detection of the antioxidant and pro-oxidant activity of dietary polyphenolics in a peroxidase system. Free Radic Res 37:787–794. https://doi.org/10.1080/1071576031000094899
Cheng CJ, Ferruzzi M, Jones OG (2019) Fate of lutein-containing zein nanoparticles following simulated gastric and intestinal digestion. Food Hydrocoll 87:229–236. https://doi.org/10.1016/j.foodhyd.2018.08.013
Cheong AM, Tan KW, Tan CP, Nyam KL (2016) Kenaf (Hibiscus cannabinus L.) seed oil-in-water Pickering nanoemulsions stabilised by mixture of sodium caseinate, tween 20 and β-cyclodextrin. Food Hydrocoll 52:934–941. https://doi.org/10.1016/j.foodhyd.2015.09.005
Chikwere P (2017) Functional foods and nutraceuticals, wonders in cancer risks—a review. World Sci News 64:18–33
CAS Google Scholar
Choi SUS, Eastman JA (1995) Enhancing thermal conductivity of fluids with nanoparticles. In: Enhancing thermal conductivity of fluids with nanoparticles (conference) | OSTI.GOV. https://www.osti.gov/biblio/196525/ . Accessed 3 Oct 2023
Chris OU, Singh NB, Agarwal A (2018) Nanoparticles as feed supplement on growth behaviour of cultured catfish (Clarias gariepinus) fingerlings. Appl Mater Today 5:9076–9081
Cohen Y, Levi M, Lesmes U et al (2017) Re-assembled casein micelles improve in vitro bioavailability of vitamin D in a Caco-2 cell model. Food Funct 8:2133–2141. https://doi.org/10.1039/c7fo00323d
Constantinescu-Aruxandei D, Frîncu R, Capră L, Oancea F (2018) Selenium analysis and speciation in dietary supplements based on next-generation selenium ingredients. Nutrients 10:1466. https://doi.org/10.3390/nu10101466
Dai L, Wei Y, Sun C et al (2018) Development of protein-polysaccharide-surfactant ternary complex particles as delivery vehicles for curcumin. Food Hydrocoll 85:75–85. https://doi.org/10.1016/j.foodhyd.2018.06.052
Dan N (2016) Compound release from nanostructured lipid carriers (NLCS). J Food Eng 171:37–43. https://doi.org/10.1016/j.jfoodeng.2015.10.005
David S, Livney YD (2016) Potato protein based nanovehicles for health promoting hydrophobic bioactives in clear beverages. Food Hydrocoll 57:229–235. https://doi.org/10.1016/j.foodhyd.2016.01.027
De Angelis F, Pujia A, Falcone C, Iaccino E, Palmieri C, Liberale C et al (2010) Water soluble nanoporous nanoparticle for in vivo targeted drug delivery and controlled release in B cells tumor context. Nanoscale 2:2230–2236. https://doi.org/10.1039/c0nr00161a
de Farias SS, Siqueira SM, Cunha AP et al (2018) Microencapsulation of riboflavin with galactomannan biopolymer and F127: physico-chemical characterization, antifungal activity and controlled release. Ind Crop Prod 118:271–281. https://doi.org/10.1016/j.indcrop.2018.03.039
Debski B (2016) Supplementation of pigs diet with zinc and copper as alternative to conventional antimicrobials. Pol J Vet Sci 19:917–924
Dey TK, Banerjee P, Chatterjee R, Dhar P (2018) Designing of ω-3 PUFA enriched biocompatible nanoemulsion with sesame protein isolate as a natural surfactant: focus on enhanced shelf-life stability and biocompatibility. Colloids Surf A Physicochem Eng Asp 538:36–44. https://doi.org/10.1016/j.colsurfa.2017.10.066
Dhull SB, Punia S (2020a) Sources. Essential Fatty. pp. 19–56. : https://doi.org/10.1201/9780429321115-2
Dhull SB, Punia S (2020b) Essential fatty acids. Essential Fatty. pp. 1–18. https://doi.org/10.1201/9780429321115-1
Dhull SB, Punia S, Sandhu KS (2020) Essential fatty acids. https://doi.org/10.1201/9780429321115
Ding L, Liu Z, Aggrey M et al (2015) Nanotoxicity: the toxicity research progress of metal and metal- containing nanoparticles. Mini-Rev Med Chem 15:529–542. https://doi.org/10.2174/138955751507150424104334
Domínguez Rubio AP, Martínez JH, Martínez Casillas DC et al (2017) Lactobacillus casei BL23 produces microvesicles carrying proteins that have been associated with its probiotic effect. Front Microbiol 8. https://doi.org/10.3389/fmicb.2017.01783
Duffy C, O’Riordan D, O’Sullivan M, Jacquier JC (2018) In vitro evaluation of chitosan copper chelate gels as a multimicronutrient feed additive for cattle. J Sci Food Agric 98:4177–4183
Durán N, Marcato PD (2013) Nanobiotechnology perspectives. Role of nanotechnology in the food industry: a review. Int J Food Sci Technol 48:1127–1134. https://doi.org/10.1111/ijfs.12027
ECHA (2021) Regulation—ECHA. https://euon.echa.europa.eu/regulation . Accessed 5 Nov 2021
EI-Sherbiny M, Cieslak A, Szczechowiak J, Kolodziejski P, Szulc P, Szumacher-Strabel M (2016) Effect of nanoemulsified oils addition on rumen fermentation and fatty acid proportion in a rumen simulation technique. J Anim Feed Sci 25:116–124
El Basuini MF, El-Hais AM, Dawood MAO, Abou-Zeid AES, EL-Damrawy SZ, Khalafalla MMES, Koshio S, Ishikawa M, Dossou S (2017) Effects of dietary copper nanoparticles and vitamin C supplementations on growth performance, immune response and stress resistance of red sea bream, Pagrus major. Aquac Nutr 23:1329–1340
El-Far A, Al Jaouni S, Li W, Mousa S (2018) Protective roles of thymoquinone nanoformulations: potential nanonutraceuticals in human diseases. Nutrients 10:1369. https://doi.org/10.3390/nu10101369
El-Shibiny S, Abd El-Gawad MAM, Assem FM, El-Sayed SM (2018) The use of nano-sized eggshell powder for calcium fortification of cow’s and buffalo’s milk yogurts. Acta Sci Pol Technol Aliment 17:37–49
CAS PubMed Google Scholar
Erfanian A, Mirhosseini H, Abd Manap MY, Rasti B, Hair-Bejo M (2014) Influence of nano-size reduction on absorption and bioavailability of calcium from fortified milk powder in rats. Food Res Int 66:1–11
Erfanian A, Mirhosseini H, Rasti B, Hair-Bejo M, Bin Mustafa S, Abd Manap MY (2015) Absorption and bioavailability of nano-size reduced calcium citrate fortified milk powder in ovariectomized and ovariectomized-osteoporosis rats. J Agric Food Chem 63:5795–57804
Erfanian A, Rasti B, Manap Y (2017) Comparing the calcium bioavailability from two types of nano-sized enriched milk using in-vivo assay. Food Chem 214:606–613
Espitia PJP, Otoni CG (2018) Nanotechnology and edible films for food packaging applications. Bio-based mater. Food Packag Green Sustain Adv Packag Mater 6:125–145. https://doi.org/10.1007/978-981-13-1909-9_6
Faridi Esfanjani A, Assadpour E, Jafari SM (2018) Improving the bioavailability of phenolic compounds by loading them within lipid-based nanocarriers. Trends Food Sci Technol 76:56–66. https://doi.org/10.1016/j.tifs.2018.04.002
Favero MS (1999) Prevention and control of nosocomial infections, 3rd Ed, RP Wenzel; Baltimore MD: Lippincott Williams & Wilkins; 1997; 1,000 pages. Infect Control Hosp Epidemiol 20:834–834. https://doi.org/10.1017/s0195941700073069
FDA (2021) Nanotechnology fact sheet. Food and Drug Administartion. https://www.fda.gov/science-research/nanotechnologyprograms-fda/nanotechnology-fact-sheet . Accessed 5 Nov 2021
Feng T, Wang K, Liu FF, Ye R, Zhu X, Zhuang HN, Xue ZM (2017) Structural characterization and bioavailability of ternary nanoparticles consisting of amylose, α-linoleic acid and β-lactoglobulin complexed with naringin. Int J Biol Macromol 99:365–374
Fritz H, Seely D, Flower G, Skidmore B, Fernandes R, Vadeboncoeur S, Kennedy D, Cooley K, Wong R, Sagar S et al (2013) Soy, red clover, and isoflavones and breast cancer: a systematic review. PLoS One 8:e81968
Galati G (2002) Prooxidant activity and cellular effects of the phenoxyl radicals of dietary flavonoids and other polyphenolics. Toxicology 177:91–104. https://doi.org/10.1016/s0300-483x(02)00198-1
Gangadoo S, Stanley D, Hughes RJ, Moore RJ, Chapman J (2016) Nanoparticles in feed: progress and prospects in poultry research. Trends Food Sci Technol 58:115–126
Gbassi GK, Vandamme T (2012) Probiotic encapsulation technology: from microencapsulation to release into the gut. Pharmaceutics 4:149–163. https://doi.org/10.3390/pharmaceutics4010149
Ge J, Yue P, Chi J et al (2018) Formation and stability of anthocyanins-loaded nanocomplexes prepared with chitosan hydrochloride and carboxymethyl chitosan. Food Hydrocoll 74:23–31. https://doi.org/10.1016/j.foodhyd.2017.07.029
George Kerry R, Patra JK, Gouda S et al (2018) Benefaction of probiotics for human health: a review. J Food Drug Anal 26:927–939. https://doi.org/10.1016/j.jfda.2018.01.002
Ghanbarzadeh B, Babazadeh A, Hamishehkar H (2016) Nano-phytosome as a potential food-grade delivery system. Food Biosci 15:126–135. https://doi.org/10.1016/j.fbio.2016.07.006
Ghayour N, Hosseini SM, Eskandari MH et al (2019) Nanoencapsulation of quercetin and curcumin in casein-based delivery systems. Food Hydrocoll 87:394–403. https://doi.org/10.1016/j.foodhyd.2018.08.031
Gleeson JP, Ryan SM, Brayden DJ (2016) Oral delivery strategies for nutraceuticals: delivery vehicles and absorption enhancers. Trends Food Sci Technol 53:90–101. https://doi.org/10.1016/j.tifs.2016.05.007
Gokmen V, Mogol BA, Lumaga RB, Fogliano V, Kaplun Z, Shimoni E (2011) Development of functional bread containing nanoencapsulated ω-3 fatty acids. J Food Eng 105:585–591
González-Reza RM, Quintanar-Guerrero D, Del Real-López A et al (2018) Effect of sucrose concentration and ph onto the physical stability of β-carotene nanocapsules. LWT 90:354–361. https://doi.org/10.1016/j.lwt.2017.12.044
Gornati R, Pedretti E, Rossi F, Cappellini F, Zanella M, Olivato I, Sabbioni E, Bernardini G (2016) Zerovalent Fe Co and Ni nanoparticle toxicity evaluated on SKOV-3 and U87 cell lines. J Appl Toxicol 36:385–393
Granja A, Frias I, Neves AR, Pinheiro M, Reis S (2017) Therapeutic potential of epigallocatechin gallate nanodelivery systems. Biomed Res Int 2017:5813793
Guo HH, Hong ZA, Yi RZ (2015) Core-shell collagen peptide chelated calcium/calcium alginate nanoparticles from fish scales for calcium supplementation. J Food Sci 80:N1595–N1601
Guo C, Yin J, Chen D (2018) Co-encapsulation of curcumin and resveratrol into novel nutraceutical hyalurosomes nano-food delivery system based on oligo-hyaluronic acid-curcumin polymer. Carbohydr Polym 181:1033–1037. https://doi.org/10.1016/j.carbpol.2017.11.046
Guttoff M, Saberi AH, McClements DJ (2015) Formation of vitamin D nanoemulsion-based delivery systems by spontaneous emulsification: factors affecting particle size and stability. Food Chem 171:117–122. https://doi.org/10.1016/j.foodchem.2014.08.087
Hafner A, Lovric J, Lakoš GP, Pepić I (2014) Nanotherapeutics in the EU: an overview on current state and future directions. Int J Nanomedicine 9:1005–1023. https://doi.org/10.2147/IJN.S55359
Hasanvand E, Fathi M, Bassiri A (2018) Production and characterization of vitamin D3 loaded starch nanoparticles: effect of amylose to amylopectin ratio and sonication parameters. J Food Sci Technol 55:1314–1324. https://doi.org/10.1007/s13197-018-3042-0
Hassan FAM, Mahmoud R, El-Araby IE (2017) Growth performance, serum biochemical, economic evaluation and IL6 gene expression in growing rabbits fed diets supplemented with zinc nanoparticles. Zagazig Vet J 45:238–249. [CrossRef]
Hategekimana J, Chamba MV, Shoemaker CF et al (2015) Vitamin E nanoemulsions by emulsion phase inversion: effect of environmental stress and long-term storage on stability and degradation in different carrier oil types. Colloids Surf A Physicochem Eng Asp 483:70–80. https://doi.org/10.1016/j.colsurfa.2015.03.020
Herbert V (1994) The antioxidant supplement myth. Am J Clin Nutr 60:157–158. https://doi.org/10.1093/ajcn/60.2.157
Hilty FM, Arnold M, Hilbe M, Teleki A, Knijnenburg JT, Ehrensperger F, Hurrell RF, Pratsinis SE, Langhans W, Zimmermann MB (2010) Iron from nanocompounds containing iron and zinc is highly bioavailable in rats without tissue accumulation. Nat Nanotechnol 5:374–380
Hosnedlova B, Kepinska M, Skalickova S, Fernandez C, Ruttkay-Nedecky B, Peng QM, Baron M, Melcova M, Opatrilova R, Zidkova J et al (2018) Nano-selenium and its nanomedicine applications: a critical review. Int J Nanomedicine 13:2107–2128
Hosny KM, Banjar ZM, Hariri AH, Hassan AH (2015) Solid lipid nanoparticles loaded with iron to overcome barriers for treatment of iron deficiency anemia. Drug Des Dev Ther 9:313–320
Hossein-Nia B, Khorram S, Rezazadeh H, Safaiyan A, Ghiasi R, Tarighat-Esfanjani A (2018) The effects of natural clinoptilolite and nano-sized clinoptilolite supplementation on lipid profile, food intakes and body weight in rats with streptozotocin-induced diabetes. Adv Pharm Bull 8:211–216
Hu K, Huang XX, Gao YQ, Huang XL, Xiao H, McClements DJ (2015) Core-shell biopolymer nanoparticle delivery systems: synthesis and characterization of curcumin fortified zein-pectin nanoparticles. Food Chem 182:275–281
Hu Y, Zhang W, Ke Z et al (2017) In vitro release and antioxidant activity of Satsuma Mandarin ( citrus reticulata blanco CV. unshiu) peel flavonoids encapsulated by pectin nanoparticles. Int J Food Sci Technol 52:2362–2373. https://doi.org/10.1111/ijfs.13520
Hu F, Sun D-S, Wang K-L, Shang D-Y (2022) Nanomedicine of plant origin for the treatment of metabolic disorders. Front Bioeng Biotechnol 9. https://doi.org/10.3389/fbioe.2021.811917
Huang S, Chen JC, Hsu CW, Chang WH (2009) Effects of nano calcium carbonate and nano calcium citrate on toxicity in ICR mice and on bone mineral density in an ovariectomized mice model. Nanotechnology 20:375102
Huang X, Huang X, Gong Y et al (2016) Enhancement of curcumin water dispersibility and antioxidant activity using core–shell protein–polysaccharide nanoparticles. Food Res Int 87:1–9. https://doi.org/10.1016/j.foodres.2016.06.009
Huang X, Dai Y, Cai J et al (2017) Resveratrol encapsulation in core-shell biopolymer nanoparticles: impact on antioxidant and anticancer activities. Food Hydrocoll 64:157–165. https://doi.org/10.1016/j.foodhyd.2016.10.029
Hung AT, Leury BJ, Sabin MA, Collins CL, Dunshea FR (2014) Dietary nano-chromium tripicolinate increases feed intake and decreases plasma cortisol in finisher gilts during summer. Trop Anim Health Prod 46:1483–1489
Huq T, Fraschini C, Khan A et al (2017) Alginate based nanocomposite for microencapsulation of probiotic: effect of cellulose nanocrystal (CNC) and lecithin. Carbohydr Polym 168:61–69. https://doi.org/10.1016/j.carbpol.2017.03.032
Husen A, Jawaid M (2020) Preface. Nanomater for Agric For Appl:xxvii–xxviii. https://doi.org/10.1016/b978-0-12-817852-2.00030-5
Huwyler J, Kettiger H, Schipanski A, Wick P (2013) Engineered nanomaterial uptake and tissue distribution: from cell to organism. Int J Nanomedicine 8:3255–3269. https://doi.org/10.2147/ijn.s49770
Ismail HTH, El-Araby IE (2017) Effect of dietary zinc oxide nanoparticles supplementation on biochemical, hematological and genotoxucity parameters in rabbits. Int J Curr Adv Res 6:2108–2115
Izquierdo MS, Ghrab W, Roo J, Hamre K, Hernandez-Cruz CM, Bernardini G, Terova G, Saleh R (2017) Organic, inorganic and nanoparticles of Se, Zn and Mn in early weaning diets for gilthead seabream (Sparus aurata; Linnaeus, 1758). Aqua Res 48:2852–2867
Jacela JY, De Rouchey JM, Tokach MD, Goodband RD, Nelssen JL, Renter DG, Dritz SS (2010) Feed additives for swine: fact sheets-high dietary levels of copper and zinc for young pigs, and phytase. J Swine Health Prod 18:87–92
Jafari SM, Assadpoor E, Bhandari B, He Y (2008) Nano-particle encapsulation of fish oil by spray drying. Food Res Int 41:172–183. https://doi.org/10.1016/j.foodres.2007.11.002
Jagtiani E (2021) Advancements in nanotechnology for food science and industry. Food Front 3:56–82. https://doi.org/10.1002/fft2.104
Jampilek J, Kos J, Kralova K (2019) Potential of nanomaterial applications in dietary supplements and foods for special medical purposes. Nano 9:296. https://doi.org/10.3390/nano9020296
Jiao Z, Wang X, Yin Y et al (2018) Preparation and evaluation of a chitosan-coated antioxidant liposome containing vitamin C and folic acid. J Microencapsul 35:272–280. https://doi.org/10.1080/02652048.2018.1467509
Joubert IA, Geppert M, Ess S, Nestelbacher R, Gadermaier G, Duschl A et al (2020) Public perception and knowledge on nanotechnology: a study based on a citizen science approach. NanoImpact 17:100201. https://doi.org/10.1016/j.impact.2019.100201
Juère E, Florek J, Bouchoucha M et al (2017) In vitro dissolution, cellular membrane permeability, and anti-inflammatory response of resveratrol-encapsulated mesoporous silica nanoparticles. Mol Pharm 14:4431–4441. https://doi.org/10.1021/acs.molpharmaceut.7b00529
Kar SK, Jansman AJM, Boeren S, Kruijt L, Smits MA (2016) Protein, peptide, amino acid composition, and potential functional properties of existing and novel dietary protein sources for monogastrics. J Anim Sci 94:30–39
Katouzian I, Jafari SM (2016) Nano-encapsulation as a promising approach for targeted delivery and controlled release of vitamins. Trends Food Sci Technol 53:34–48. https://doi.org/10.1016/j.tifs.2016.05.002
Kaur K, Kaur J, Kumar R, Mehta SK (2017) Formulation and physiochemical study of α-tocopherol based oil in water nanoemulsion stabilized with non toxic, biodegradable surfactant: sodium stearoyl lactate. Ultrason Sonochem 38:570–578. https://doi.org/10.1016/j.ultsonch.2016.08.026
Khader M, Bresgen N, Eckl P (2008) Toxicological properties of thymoquinone in primary rat hepatocyte cultures. Planta Med 74. https://doi.org/10.1055/s-0028-1084326
Khan KU, Zuberi A, Nazir S, Fernandes JBK, Jamil Z, Sarwar H (2016) Effects of dietary selenium nanoparticles on physiological and biochemical aspects of juvenile tor putitora. Turk J Zool 40:704–712
Khan A, Wen Y, Huq T, Ni Y (2017) Cellulosic nanomaterials in food and nutraceutical applications: a review. J Agric Food Chem 66:8–19. https://doi.org/10.1021/acs.jafc.7b04204
Khashayar P, Keshtkar A, Ebrahimi M, Larijani B (2015) Nano calcium supplements: friends or foes? J Bone Biol Osteoporosis 1:32–33
Khorasani S, Danaei M, Mozafari MR (2018) Nanoliposome technology for the food and nutraceutical industries. Trends Food Sci Technol 79:106–115. https://doi.org/10.1016/j.tifs.2018.07.009
Kojouri GA, Jahanabadi S, Shakibaie M, Ahadi AM, Shahverdi AR (2012) Effect of selenium supplementation with sodium selenite and selenium nanoparticles on iron homeostasis and transferrin gene expression in sheep: a preliminary study. Res Vet Sci 93:275–278
Kong R, Xia Q, Liu GY (2011) Preparation and characterization of vitamin A palmitate-loaded nanostructured lipid carriers as delivery systems for food products. Adv Mater Res 236–238:1818–1823. https://doi.org/10.4028/www.scientific.net/amr.236-238.1818
Kosla T, Lasocka I, Skibniewska EM, Kolnierzak M, Skibniewski M (2018) Trivalent chromium (Cr III) as a trace element essential for animals and humans. Med Weter 74:560–567
Kumar LY (2015) Role and adverse effects of nanomaterials in food technology. J Toxicol Health 2:2. https://doi.org/10.7243/2056-3779-2-2
Kumar SA, Brown L (2018) Alginates in metabolic syndrome. In: Rehm BHA, Moradali MF (eds) Aginates and theirbiomedical applications, vol 11. Springer, Singapore, pp 223–235
Kumar N, Krishnani KK, Singh NP (2018a) Effect of dietary zinc-nanoparticles on growth performance, anti-oxidative and immunological status of fish reared under multiple stressors. Biol Trace Elem Res 186:267–278
Kumar N, Krishnani KK, Gupta SK, Sharma R, Baitha R, Singh DK, Singh NP (2018b) Immuno-protective role of biologically synthesized dietary selenium nanoparticles against multiple stressors in Pangasinodon hypophthalrnus. Fish Shellfish Immunol 78:289–298
Kumar B, Singh N, Dey S, Sahoo PK (2022) A comprehensive review on nanotechnological approaches for enhanceddelivery of nutraceuticals in the management of osteoporosis. Drug Deliv Lett 12:96–108. https://doi.org/10.2174/2210303112666220414100934
Kuo SM, Merhige PM, Hagey LR (2013) The effect of dietary prebiotics and probiotics on body weight, large intestine indices, and fecal bile acid profile in wild type and IL10−/− mice. PLoS One 8:60270
Lagaron J-M (2011) Multifunctional and nanoreinforced polymers for food packaging. https://doi.org/10.1533/9780857092786
Lee JH, Cha KE, Kim MS, Hong HW, Chung DJ, Ryu G et al (2009) Nanosized polyamidoamine (PAMAM) dendrimer-induced apoptosis mediated by mitochondrial dysfunction. Toxicol Lett 190:202–207. https://doi.org/10.1016/j.toxlet.2009.07.018
Lefranc F, Tabanca N, Kiss R (2017) Assessing the anticancer effects associated with food products and/or nutraceuticals using in vitro and in vivo preclinical development-related pharmacological tests. Sem Cancer Biol 46:14–32
Leroueil PR, Berry SA, Duthie K, Han G, Rotello VM, McNerny DQ et al (2008) Wide varieties of cationic nanoparticles induce defects in supported lipid bilayers. Nano Lett 8:420–424. https://doi.org/10.1021/nl0722929
Li X, Yin J, Li D, Chen X, Zang J, Zhou X (2006) Dietary supplementation with zinc oxide increases Igf-I and Igf-I receptor gene expression in the small intestine of weanling piglets. J Nutr 136:1786–1791
Li X, Wang L, Fan Y, Feng Q, Cui FZ (2012) Biocompatibility and toxicity of nanoparticles and nanotubes. J Nanomater 2012:548389. https://doi.org/10.1155/2012/548389
Li MZ, Huang JT, Tsai YH, Mao SY, Fu CM, Lien TF (2016) Nanosize of zinc oxide and the effects on zinc digestibility, growth performances, immune response and serum parameters of weanling piglets. Anim Sci J 87:1379–1385
Li Q, Li T, Liu C et al (2017) Enhancement of carotenoid bioaccessibility from tomatoes using excipient emulsions: influence of particle size. Food Biophysics 12:172–185. https://doi.org/10.1007/s11483-017-9474-7
Li Z, Peng S, Chen X et al (2018) Pluronics modified liposomes for curcumin encapsulation: sustained release, stability and bioaccessibility. Food Res Int 108:246–253. https://doi.org/10.1016/j.foodres.2018.03.048
Lin Y, Wang Y-H, Yang X-Q et al (2016) Corn protein hydrolysate as a novel nano-vehicle: enhanced physicochemical stability and in vitro bioaccessibility of vitamin D3. LWT Food Sci Technol 72:510–517. https://doi.org/10.1016/j.lwt.2016.05.020
Liu L, Gao YX, McClements DJ, Decker EA (2016a) Role of continuous phase protein, (−)-epigallocatechin-3-gallate and carrier oil on beta-carotene degradation in oil-in-water emulsions. Food Chem 210:242–248
Liu X, Bi J, Xiao H, McClements DJ (2016b) Enhancement of nutraceutical bioavailability using excipient nanoemulsions: role of lipid digestion products on bioaccessibility of carotenoids and phenolics from mangoes. J Food Sci 81:N754. https://doi.org/10.1111/1750-3841.13227
Liu G, Huang W, Babii O et al (2018a) Novel protein–lipid composite nanoparticles with an inner aqueous compartment as delivery systems of hydrophilic nutraceutical compounds. Nanoscale 10:10629–10640. https://doi.org/10.1039/c8nr01009a
Liu Y, Fan Y, Gao L et al (2018b) Enhanced PH and thermal stability, solubility and antioxidant activity of resveratrol by nanocomplexation with α-lactalbumin. Food Funct 9:4781–4790. https://doi.org/10.1039/c8fo01172a
Liu F, Ma D, Luo X et al (2018c) Fabrication and characterization of protein-phenolic conjugate nanoparticles for co-delivery of curcumin and resveratrol. Food Hydrocoll 79:450–461. https://doi.org/10.1016/j.foodhyd.2018.01.017
Liu YT, Zeng SG, Liu YX, Wu WJ, Shen YB, Zhang L, Li C, Chen H, Liu AP, Shen L (2018d) Synthesis and antidiabetic activity of selenium nanoparticles in the presence of polysaccharides from Catathelasma ventricosum. Int J Biol Macromol 114:632–639
Lonnerdal B, Bryant A, Liu X, Theil EC (2006) Iron absorption from soybean ferritin in nonanemic women. Am J Clin Nutr 83:103–107
Lotha R, Sivasubramanian A (2018) Flavonoids nutraceuticals in prevention and treatment of cancer: a review. Asian J Pharm Clin Res 11:42–47
Luo Y, Wang Q, Zhang Y (2020) Biopolymer-based nanotechnology approaches to deliver bioactive compounds for food applications: a perspective on the past, present, and future. J Agric Food Chem 68:12993–13000. https://doi.org/10.1021/acs.jafc.0c00277
Madalena DA, Ramos ÓL, Pereira RN et al (2016) In vitro digestion and stability assessment of β-lactoglobulin/riboflavin nanostructures. Food Hydrocoll 58:89–97. https://doi.org/10.1016/j.foodhyd.2016.02.015
Magnuson BA, Jonaitis TS, Card JW (2011) A brief review of the occurrence, use, and safety of food-related nanomaterials. J Food Sci 76:R126. https://doi.org/10.1111/j.1750-3841.2011.02170.x
Mahmoud MH, Badr G, El Shinnawy NA (2016) Camel whey protein improves lymphocyte function and protects against diabetes in the offspring of diabetic mouse dams. Int J Immunopathol Pharmacol 29:632–646
Mao SY, Lien TF (2017) Effects of nanosized zinc oxide and -polyglutamic acid on eggshell quality and serum parameters of aged laying hens. Arch Anim Nutr 71:373
Mao L, Wang D, Liu F, Gao Y (2017) Emulsion design for the delivery of β-carotene in complex food systems. Crit Rev Food Sci Nutr 58:770–784. https://doi.org/10.1080/10408398.2016.1223599
Martins ACD, Flores JA, Porto C, Romano LA, Wasielesky JW, Caldas SS, Primel EG, Kulkamp-Guerreiro I, Monserrat JM (2018) Antioxidant effects of nanoencapsulated lipoic acid in tissues and on the immune condition in haemolymph of Pacific white shrimp Litopenaeus vannamei (Boone, 1931). Aquac Nutr 24:1255–1262
McClements D, Öztürk B (2021) Utilization of nanotechnology to improve the handling, storage and biocompatibility of bioactive lipids in food applications. Food Secur 10:365. https://doi.org/10.3390/foods10020365
McClements DJ, Xiao H (2017) Designing food structure and composition to enhance nutraceutical bioactivity to support cancer inhibition. Semin Cancer Biol 46:215–226
Meenambal R, Srinivas Bharath MM (2020) Nanocarriers for effective nutraceutical delivery to the brain. Neurochem Int 140:104851. https://doi.org/10.1016/j.neuint.2020.104851
Meghani N, Patel P, Kansara K, Ranjan S, Dasgupta N, Ramalingam C, Kumar A (2018) Formulation of vitamin D encapsulated cinnamon oil nanoemulsion: its potential anti-cancerous activity in human alveolar carcinoma cells. Colloids Surf B Biointerface 166:349–357
Mijan MA, Lee YK, Kwak HS (2014) Effects of nanopowdered eggshell on postmenopausal osteoporosis: a rat study. Food Sci Biotechnol 23:1667–1676
Mishra SS, Behera PK, Kar B, Ray RC (2018a) Advances in probiotics, prebiotics and nutraceuticals. In: Panda S, Shetty P (eds) Innovations in technologies for fermented food and beverage industries. Springer, Cham, pp 121–141
Chapter Google Scholar
Mishra G, Dash B, Pandey S (2018b) Layered double hydroxides: a brief review from fundamentals to application as evolving biomaterials. Appl Clay Sci 153:172–186. https://doi.org/10.1016/j.clay.2017.12.021
Mitura KA, Zarzycki PK (2018) Biocompatibility and toxicity of allotropic forms of carbon in food packaging. Role Mater Sci Food Bioeng:73–107. https://doi.org/10.1016/b978-0-12-811448-3.00003-6
Moeller H, Martin D, Schrader K et al (2018) Spray- or freeze-drying of casein micelles loaded with vitamin D2: studies on storage stability and in vitro digestibility. LWT 97:87–93. https://doi.org/10.1016/j.lwt.2018.04.003
Naahidi S, Jafari M, Edalat F et al (2013) Biocompatibility of engineered nanoparticles for drug delivery. J Control Release 166:182–194. https://doi.org/10.1016/j.jconrel.2012.12.013
Naderi M, Keyvanshokooh S, Salati AP, Ghaedi A (2017) Combined or individual effects of dietary vitamin E and selenium nanoparticles on humoral immune status and serum parameters of rainbow trout (Oncorhynchus mykiss) under high stocking density. Aquaculture 474:40–47
Nagarajan S, Soussan L, Bechelany M et al (2016) Novel biocompatible electrospun gelatin fiber mats with antibiotic drug delivery properties. J Mater Chem B 4:1134–1141. https://doi.org/10.1039/c5tb01897h
Nakada H, Sakae T, Watanabe T, Takahashi T, Fujita K, Tanimoto Y, Teranishi M, Kato T, Kawai Y (2014) A new osteoporosis prevention supplements-diet improve bone mineral density in ovariectomized rats on micro-CT. J Hard Tissue Biol 23:1–8
Nia BH, Khorram S, Rezazadeh H, Safaiyan A, Tarighat-Esfanjani A (2018) The effects of natural clinoptilolite and nano-sized clinoptilolite supplementation on glucose levels and oxidative stress in rats with type 1 diabetes. Can J Diabetes 42:31–35
Nikonov IN, Folmanis YG, Folmanis GE, Kovalenko LV, Laptev GY, Egorov IA, Fisinin VI, Tananaev IG (2011) Iron nanoparticles as a food additive for poultry. Dokl Biol Sci 440:328–331
Noor Z (2013) Nanohydroxyapatite application to osteoporosis management. J Osteoporos 2013:679025
Nunes S, Madureira R, Campos D et al (2015) Solid lipid nanoparticles as oral delivery systems of phenolic compounds: overcoming pharmacokinetic limitations for nutraceutical applications. Crit Rev Food Sci Nutr:00. https://doi.org/10.1080/10408398.2015.1031337
Ochnio M, Martínez J, Allievi M et al (2018) Proteins as nano-carriers for bioactive compounds. The case of 7s and 11s soy globulins and folic acid complexation. Polymers 10:149. https://doi.org/10.3390/polym10020149
Ognik K, Sembratowicz I, Cholewinska E, Jankowski J, Kozlowski K, Juskiewicz J, Zdunczyk Z (2018) The effect of administration of copper nanoparticles to chickens in their drinking water on the immune and antioxidant status of the blood. Anim Sci J 89:579–588
Ozturk B, Argin S, Ozilgen M, McClements DJ (2014) Formation and stabilization of nanoemulsion-based vitamin E delivery systems using natural surfactants: Quillaja saponin and lecithin. J Food Eng 142:57–63. https://doi.org/10.1016/j.jfoodeng.2014.06.015
Pagano C, Tiralti MC, Perioli L (2016) Nanostructured hybrids for the improvement of folic acid biopharmaceutical properties. J Pharm Pharmacol 68:1384–1395. https://doi.org/10.1111/jphp.12634
Pandey KR, Naik SR, Vakil BV (2015) Probiotics, prebiotics and synbiotics- a review. J Food Sci Technol 52:7577–7587. https://doi.org/10.1007/s13197-015-1921-1
Papagiannopoulos A, Vlassi E (2019) Stimuli-responsive nanoparticles by thermal treatment of bovine serum albumin inside its complexes with chondroitin sulfate. Food Hydrocoll 87:602–610. https://doi.org/10.1016/j.foodhyd.2018.08.054
Park JH, Gu L, Von Maltzahn G, Ruoslahti E, Bhatia SN, Sailor MJ (2009) Biodegradable luminescent porous silicon nanoparticles for in vivo applications. Nat Mater 8:331–336. https://doi.org/10.1038/nmat2398
Parthasarathi S, Anandharamakrishnan C (2016) Enhancement of oral bioavailability of vitamin E by spray-freeze drying of whey protein microcapsules. Food Bioprod Process 100:469–476. https://doi.org/10.1016/j.fbp.2016.09.004
Parthasarathi S, Muthukumar SP, Anandharamakrishnan C (2016) The influence of droplet size on the stability, in vivo digestion, and oral bioavailability of vitamin E emulsions. Food Funct 7:2294–2302. https://doi.org/10.1039/c5fo01517k
Paucar OC, Tulini FL, Thomazini M et al (2016) Production by spray chilling and characterization of solid lipid microparticles loaded with vitamin D 3. Food Bioprod Process 100:344–350. https://doi.org/10.1016/j.fbp.2016.08.006
Paul D, Dey TK, Mukherjee S, Ghosh M, Dhar P (2014) Comparative prophylactic effects of alpha-eleostearic acid rich nano and conventional emulsions in induced diabetic rats. J Food Sci Tech Mysore 51:1724–1736
Penalva R, Esparza I, Agüeros M et al (2015) Casein nanoparticles as carriers for the oral delivery of folic acid. Food Hydrocoll 44:399–406. https://doi.org/10.1016/j.foodhyd.2014.10.004
Peng S, Li Z, Zou L et al (2018a) Improving curcumin solubility and bioavailability by encapsulation in saponin-coated curcumin nanoparticles prepared using a simple ph-driven loading method. Food Funct 9:1829–1839. https://doi.org/10.1039/c7fo01814b
Peng S, Li Z, Zou L et al (2018b) Enhancement of curcumin bioavailability by encapsulation in sophorolipid-coated nanoparticles: an in vitro and in vivo study. J Agric Food Chem 66:1488–1497. https://doi.org/10.1021/acs.jafc.7b05478
Peredo AG, Beristain CI, Pascual LA, Azuara E, Jimenez M (2016) The effect of prebiotics on the viability of encapsulated probiotic bacteria. Food Sci Technol 73:191–196
Pereira DIA, Bruggraber SFA, Faria N, Poots LK, Tagmount MA, Aslam MF, Frazer DM, Vulpe CD, Anderson GJ, Powell JJ (2014) Nanoparticulate iron(III) oxo-hydroxide delivers safe iron that is well absorbed and utilised in humans. Nanomedicine 10:1877–1886
Pereira DIA, Mohammed NI, Ofordile O, Camara F, Baldeh B, Mendy T, Sanyang C, Jallow AT, Hossain I, Wason J et al (2018) A novel nano-iron supplement to safely combat iron deficiency and anaemia in young children: the IHAT-GUT double-blind, randomised, placebo-controlled trial protocol. Gates Open Res 2:48
Pérez-Esteve É, Fuentes A, Coll C et al (2015) Modulation of folic acid bioaccessibility by encapsulation in ph-responsive gated mesoporous silica particles. Microporous Mesoporous Mater 202:124–132. https://doi.org/10.1016/j.micromeso.2014.09.049
Pérez-Esteve É, Ruiz-Rico M, de la Torre C et al (2016a) Encapsulation of folic acid in different silica porous supports: a comparative study. Food Chem 196:66–75. https://doi.org/10.1016/j.foodchem.2015.09.017
Pérez-Esteve É, Ruiz-Rico M, Fuentes A et al (2016b) Enrichment of stirred yogurts with folic acid encapsulated in ph-responsive mesoporous silica particles: bioaccessibility modulation and physico-chemical characterization. LWT Food Sci Technol 72:351–360. https://doi.org/10.1016/j.lwt.2016.04.061
Perurnal V, Manickam T, Bang KS, Velmurugan P, Oh BT (2016) Antidiabetic potential of bioactive molecules coated chitosan nanoparticles in experimental rats. Int J Biol Macromol 92:63–69
Pham TM, Ekwaru JP, Mastroeni SS, Mastroeni MF, Loehr SA, Veugelers PJ (2016) The effect of serum 25-hydroxyvitamin D on elevated homocysteine concentrations in participants of a preventive health program. PLoS One 11:0161368
Pisoschi AM, Pop A, Cimpeanu C et al (2018) Nanoencapsulation techniques for compounds and products with antioxidant and antimicrobial activity—a critical view. Eur J Med Chem 157:1326–1345. https://doi.org/10.1016/j.ejmech.2018.08.076
Pizzino G, Irrera N, Cucinotta M et al (2017) Oxidative stress: harms and benefits for human health. Oxidative Med Cell Longev 2017:1–13. https://doi.org/10.1155/2017/8416763
Ponzoni A, Comini E, Concina I et al (2012) Nanostructured metal oxide gas sensors, a survey of applications carried out at sensor lab, Brescia (Italy) in the security and food quality fields. Sensors 12:17023–17045. https://doi.org/10.3390/s121217023
Powell JJ, Bruggraber SFA, Faria N, Poots LK, Hondow N, Pennycook TJ, Latunde-Dada GO, Simpson RJ, Brown AP, Pereira DIA (2014) A nano-disperse ferritin-core mimetic that efficiently corrects anemia without luminal iron redox activity. Nanomedicine 10:1529–1538
Qin FJ, Shi MM, Yuan HX, Yuan LX, Lu WH, Zhang J, Tong J, Song XH (2016) Dietary nano-selenium relieves hypoxia stress and, improves immunity and disease resistance in the Chinese mitten crab (Eriocheir sinensis). Fish Shellfish Immunol 54:481–488
Qiu C, Wang C, Gong C et al (2020) Advances in research on preparation, characterization, interaction with proteins, digestion and delivery systems of starch-based nanoparticles. Int J Biol Macromol 152:117–125. https://doi.org/10.1016/j.ijbiomac.2020.02.156
Quagliariello V, Vecchione R, Coppola C, Di Cicco C, De Capua A, Piscopo G, Paciello R, Narciso V, Formisano C, Taglialatela-Scafati O et al (2018) Cardioprotective effects of nanoemulsions loaded with anti-inflammatory nutraceuticals against doxorubicin-induced cardiotoxicity. Nutrients 10:1304
Rahmatollah D, Farzinpour A, Vaziry A, Sadeghi G (2018) Effect of replacing dietary FeSO4 with cysteine-coated Fe3O4 nanoparticles on quails. Ital J Anim Sci 17:121–127. [CrossRef]
Ramalingam P, Yoo SW, Ko YT (2016) Nanodelivery systems based on mucoadhesive polymer coated solid lipid nanoparticles to improve the oral intake of food curcumin. Food Res Int 84:113–119. https://doi.org/10.1016/j.foodres.2016.03.031
Ramos OL, Pereira RN, Martins A et al (2017) Design of whey protein nanostructures for incorporation and release of nutraceutical compounds in food. Crit Rev Food Sci Nutr 57:1377–1393. https://doi.org/10.1080/10408398.2014.993749
Ranjha MM, Shafique B, Rehman A et al (2022) Biocompatible nanomaterials in food science, technology, and nutrient drug delivery: recent developments and applications. Front Nutr 8:778155. https://doi.org/10.3389/fnut.2021.778155
Ranzoni A, Cooper MA (2017) The growing influence of nanotechnology in our lives. Micro Nanotechnol Vaccine Dev 4:1–20. https://doi.org/10.1016/B978-0-323-39981-4.00001-4
Refaie AM, Ghazal MN, Easa FM, Barakat SA, Morsy WA, Younan GE, Eisa WH (2015) Nano-copper as a new growth promoter in the diet of growing New Zealand white rabbits. Egypt J Rabbit Sci 25:39–57
Rezaei A, Fathi M, Jafari SM (2019) Nanoencapsulation of hydrophobic and low-soluble food bioactive compounds within different nanocarriers. Food Hydrocoll 88:146–162. https://doi.org/10.1016/j.foodhyd.2018.10.003
Rigacci S, Stefani M (2015) Nutraceuticals and amyloid neurodegenerative diseases: a focus on natural phenols. Expert Rev Neurother 1:41–52
Robinson NG (2018) Nutraceuticals and dietary supplements. MSD Veterinary Manual
Rohani SM, Haghighi M, Moghaddam BS (2017) Study on nanoparticles of Aloe vera extract on growth performance, survival rate and body composition in Siberian sturgeon (Acipenser baerii). Iran J Fish Sci 16:457–468
Ruiz-Carrasco K, Antognoni F, Coulibaly AK et al (2011) Variation in salinity tolerance of four lowland genotypes of quinoa (chenopodium quinoa willd.) as assessed by growth, physiological traits, and sodium transporter gene expression. Plant Physiol Biochem 49:1333–1341. https://doi.org/10.1016/j.plaphy.2011.08.005
Ruiz-Rico M, Pérez-Esteve É, Lerma-García MJ et al (2017) Protection of folic acid through encapsulation in mesoporous silica particles included in fruit juices. Food Chem 218:471–478. https://doi.org/10.1016/j.foodchem.2016.09.097
Saffari S, Keyvanshokooh S, Zakeri M, Johari SA, Pasha-Zanoosi H, Mozanzadeh MT (2018) Effects of dietary organic, inorganic, and nanoparticulate selenium sources on growth, hemato-immunological, and serum biochemical parameters of common carp (Cyprinus carpio). Fish Physiol Biochem 44:1087–1097
Saha U, Fayiga A, Hancock D, Sonon L (2016) Selenium in animal nutrition: deficiencies in soils and forages, requirements, supplementation and toxicity. Int J Appl Agric Sci 2:112–125
Sajid M, Ilyas M, Basheer C et al (2014) Impact of nanoparticles on human and environment: review of toxicity factors, exposures, control strategies, and future prospects. Environ Sci Pollut Res 22:4122–4143. https://doi.org/10.1007/s11356-014-3994-1
Salaheldin TA, Regheb EM (2016) In-vivo nutritional and toxicological evaluation of nano iron fortified biscuits as food supplement for iron deficient anemia. J Nanomed Res 3:00049. Nanomaterials, 9, 296 39 of 42
Salami A, Seydi E, Pourahmad J (2013) Use of nutraceuticals for prevention and treatment of cancer. Iran J Pharm Res 12:219–220
PubMed PubMed Central Google Scholar
Salvia-Trujillo L, McClements DJ (2016) Improvement of β-carotene bioaccessibility from dietary supplements using excipient nanoemulsions. J Agric Food Chem 64:4639–4647. https://doi.org/10.1021/acs.jafc.6b00804
Sampathkumar K, Loo SC (2018) Targeted gastrointestinal delivery of nutraceuticals with polysaccharide-based coatings. Macromol Biosci 18:e1700363. https://doi.org/10.1002/mabi.201700363
Sanoj Rejinold N, Muthunarayanan M, Divyarani VV, Sreerekha PR, Chennazhi KP, Nair SV et al (2011) Curcumin-loaded biocompatible thermoresponsive polymeric nanoparticles for cancer drug delivery. J Colloid Interface Sci 360:39–51. https://doi.org/10.1016/j.jcis.2011.04.006
Sathyabama S, Kumar MR, Devi PB, Vijayabharathi R, Priyadharisini VB (2014) Co-encapsulation of probiotics with prebiotics on alginate matrix and its effect on viability in simulated gastric environment. Food Sci Technol 57:419–425
Sawosz F, Pineda L, Hotowy A, Jaworski S, Prasek M, Sawosz E, Chwalibog A (2013) Nano-nutrition of chicken embryos. The effect of silver nanoparticles and ATP on expression of chosen genes involved in myogenesis. Arch Anim Nutr 67:347–355
Saxena V, Hasan A, Sharma S, Pandey LM (2017) Edible oil nanoemulsion: an organic nanoantibiotic as a potential biomolecule delivery vehicle. Int J Polym Mater Polym Biomater 67:410–419. https://doi.org/10.1080/00914037.2017.1332625
Sayed E, Haj-Ahmad R, Ruparelia K et al (2017) Porous inorganic drug delivery systems—a review. AAPS PharmSciTech 18:1507–1525. https://doi.org/10.1208/s12249-017-0740-2
Scott A, Vadalasetty KP, Lukasiewicz M, Jaworski S, Wierzbicki M, Chwalibog A, Sawosz E (2018) Effect of different levels of copper nanoparticles and copper sulphate on performance, metabolism and blood biochemical profiles in broiler chicken. J Anim Physiol Anim Nutr (Berl) 102:E364–E373
Sechi M, Syed DN, Pala N, Mariani A, Marceddu S, Brunetti A, Mukhtar H, Sanna V (2016) Nanoencapsulation of dietary flavonoid fisetin: formulation and in vitro antioxidant and α-glucosidase inhibition activities. Mater Sci Eng C Mater Biol Appl 68:594–602
Semenova MG, Antipova AS, Zelikina DV et al (2016) Biopolymer nanovehicles for essential polyunsaturated fatty acids: structure–functionality relationships. Food Res Int 88:70–78. https://doi.org/10.1016/j.foodres.2016.05.008
Severin AV, Mazina SE, Melikhov IV (2009) Physicochemical aspects of the antiseptic action of nanohydroxyapatite. Biophysics 54:701–705
Shaphar Z, Johari SA (2018) Effects of dietary organic, inorganic, and nanoparticulate zinc on rainbow trout, vol 190. Biol Trace Elem Res, Oncorhynchus mykiss larvae, pp 535–540
Sharifi F, Jahangiri M (2018) Investigation of the stability of vitamin D in emulsion-based delivery systems. Chem Ind Chem Eng Q 24:157–167. https://doi.org/10.2298/ciceq160408028s
Shi LG, Xun WJ, Yue WB, Zhang CX, Ren YS, Liu QA, Wang QA, Shi L (2011) Effect of elemental nano-selenium on feed digestibility, rumen fermentation, and purine derivatives in sheep. Anim Feed Sci Technol 163:136–142
Shinde A, Ganu J, Naik P (2012) Effect of free radicals and antioxidants on oxidative stress: a review. J Dent Allied Sci 1:63. https://doi.org/10.4103/2277-4696.159144
Siegrist S, Cörek E, Detampel P, Sandström J, Wick P, Huwyler J (2019) Preclinical hazard evaluation strategy for nanomedicines. Nanotoxicology 13:73–99. https://doi.org/10.1080/17435390.2018.1505000
Silva HD, Poejo J, Pinheiro AC et al (2018) Evaluating the behaviour of curcumin nanoemulsions and multilayer nanoemulsions during dynamic in vitro digestion. J Funct Foods 48:605–613. https://doi.org/10.1016/j.jff.2018.08.002
Singh S, Rathi N, Angal A et al (2016) Biofortification of food with minerals and vitamins encapsulated in silica. Sustain Agric Rev:157–206. https://doi.org/10.1007/978-3-319-39306-3_5
Skibinski CG, Das A, Chen KM, Liao J, Manni A, Kester M, El-Bayoumy K (2016) A novel biologically active acid stable liposomal formulation of docosahexaenoic acid in human breast cancer cell lines. Chem Biol Interact 252:1–8
Song ZG, Lv JD, Sheikhahmadi A, Uerlings J (2017) Everaert, N. Attenuating effect of zinc and vitamin E on the intestinal oxidative stress induced by silver nanoparticles in broiler chickens. Biol Trace Elem Res 180:306–313
Srinivasu BY, Mitra G, Muralidharan M, Srivastava D, Pinto J, Thankachan P, Suresh S, Shet A, Rao S, Ravikumar G et al (2015) Beneficiary effect of nanosizing ferric pyrophosphate as food fortificant in iron deficiency anemia: evaluation of bioavailability, toxicity and plasma biomarker. RSC Adv 5:61678–61687
Summerlin N, Qu Z, Pujara N et al (2016) Colloidal mesoporous silica nanoparticles enhance the biological activity of resveratrol. Colloids Surf B: Biointerfaces 144:1–7. https://doi.org/10.1016/j.colsurfb.2016.03.076
Sun Q, Zhang Z, Zhang R et al (2018) Development of functional or medical foods for oral administration of insulin for diabetes treatment: Gastroprotective edible microgels. J Agric Food Chem 66:4820–4826. https://doi.org/10.1021/acs.jafc.8b00233
Sun T, Shrike Zhang Y, Bo P, et al. (2021) Engineered nanoparticles for drug delivery in cancer therapy. Nanomaterials and neoplasms, pp. 31–142. https://doi.org/10.1201/9780429027819-2
Swain PS, Rajendran D, Rao SB, Dominic G (2015) Preparation and effects of nano mineral particle feeding in livestock: a review. Vet World 2(8):888–891
Swain PS, Rao SBN, Rajendran D, Dominic G, Selvaraju S (2016) Nano zinc, an alternative to conventional zinc as animal feed supplement: a review. Anim Nutr 2:134–141
Tamjidi F, Shahedi M, Varshosaz J, Nasirpour A (2017a) Stability of Astaxanthin-loaded nanostructured lipid carriers as affected by ph, ionic strength, heat treatment, simulated gastric juice and freeze–thawing. J Food Sci Technol 54:3132–3141. https://doi.org/10.1007/s13197-017-2749-7
Tamjidi F, Shahedi M, Varshosaz J, Nasirpour A (2017b) Stability of astaxanthin-loaded nanostructured lipid carriers in beverage systems. J Sci Food Agric 98:511–518. https://doi.org/10.1002/jsfa.8488
Tapia-Hernández JA, Rodríguez-Felix F, Juárez-Onofre JE et al (2018) Zein-polysaccharide nanoparticles as matrices for antioxidant compounds: a strategy for prevention of chronic degenerative diseases. Food Res Int 111:451–471. https://doi.org/10.1016/j.foodres.2018.05.036
Tarighat-Esfanjani A, Fallahnejad H, Omidi H, Jafarabadi MA, Abbasi MM, Khorram S (2018) The effects of natural nano-sized clinoptilolite and metformin on the levels of serum glucose, lipid profile, and minerals in rats with type 2 diabetes mellitus. Iran Red Crescent Med J 20:74365
Tavakoli H, Hosseini O, Jafari SM, Katouzian I (2018) Evaluation of physicochemical and antioxidant properties of yogurt enriched by olive leaf phenolics within nanoliposomes. J Agric Food Chem 66:9231–9240. https://doi.org/10.1021/acs.jafc.8b02759
Torres-Giner S, Martinez-Abad A, Ocio MJ, Lagaron JM (2010) Stabilization of a nutraceutical omega-3 fatty acid by encapsulation in ultrathin electrosprayed zein prolamine. J Food Sci 75:N69. https://doi.org/10.1111/j.1750-3841.2010.01678.x
Trofimov A, Ivanova A, Zyuzin M, Timin A (2018) Porous inorganic carriers based on silica, calcium carbonate and calcium phosphate for controlled/modulated drug delivery: fresh outlook and future perspectives. Pharmaceutics 10:167. https://doi.org/10.3390/pharmaceutics10040167
Tsai YH, Mao SY, Li MZ, Huang JT, Lien TF (2016) Effects of nanosize zinc oxide on zinc retention, eggshell quality, immune response and serum parameters of aged laying hens. Anim Feed Sci Technol 213:99–107
US EPA (2015) Documentation for greenhouse gas emission and energy factors used in the waste reduction model (WARM) containers, packaging, and non-durable good materials chapters. U.S. Environmental Protection Agency, Washington, DC
Valpotic H, Gracner D, Turk R, Duricic D, Vince S, Folnozic I, Lojkic M, Zaja IZ, Bedrica L, Macesic N et al (2017) Zeolite clinoptilolite nanoporous feed additive for animals of veterinary importance: potentials and limitations. Period Biol 119:159–172
Wang MQ, Xu ZR, Zha LY, Lindemann MD (2007) Effects of chromium nanocomposite supplementation on blood metabolites, endocrine parameters and immune traits in finishing pigs. Anim Feed Sci Technol 139:69–80
Wang Z, Ma G, Zhang J et al (2014a) Development of zwitterionic polymer-based doxorubicin conjugates: tuning the surface charge to prolong the circulation and reduce toxicity. Langmuir 30:3764–3774. https://doi.org/10.1021/la5000765
Wang S, Su R, Nie S et al (2014b) Application of nanotechnology in improving bioavailability and bioactivity of diet-derived phytochemicals. J Nutr Biochem 25:363–376. https://doi.org/10.1016/j.jnutbio.2013.10.002
Wang MQ, Wang C, Du YJ, Li H, Tao WJ, Ye SS, He YD, Chen SY (2014c) Effects of chromium-loaded chitosan nanoparticles on growth, carcass characteristics, pork quality, and lipid metabolism in finishing pigs. Livest Sci 161:123–129
Wang W, Sun C, Mao L et al (2016) The biological activities, chemical stability, metabolism and delivery systems of quercetin: a review. Trends Food Sci Technol 56:21–38. https://doi.org/10.1016/j.tifs.2016.07.004
Wang H, Zhu HY, Wang XD, Li EC, Du ZY, Qin JG, Chen LQ (2018) Comparison of copper bioavailability in copper-methionine, nano-copper oxide and copper sulfate additives in the diet of Russian sturgeon Acipenser gueldenstaedtii. Aquaculture 482:146–154
Wargovich MJ, Morris J, Brown V, Ellis J, Logothetis B, Weber R (2010) Nutraceutical use in late-stage cancer. Cancer Metastasis Rev 29:503–510
Weng X, Neethirajan S (2017) Ensuring food safety: quality monitoring using microfluidics. Trends Food Sci Technol 65:10–22. https://doi.org/10.1016/j.tifs.2017.04.015
World Health Organization (2010) FAO/WHO expert meeting on the application of nanotechnologies in the food and agriculture sectors: potential food safety implications: meeting report. https://www.who.int/publications/i/item/9789241563932 . Accessed 3 Oct 2023
Nanowerk (n.d.) Nanotechnology in food. https://www.nanowerk.com/nanotechnology-in-food.php . Accessed 3 Nov 2021
Xia S, Tan C, Xue J et al (2013) Chitosan/tripolyphosphate-nanoliposomes core-shell nanocomplexes as vitamin e carriers: shelf-life and thermal properties. Int J Food Sci Technol 49:1367–1374. https://doi.org/10.1111/ijfs.12438
Xie CL, Lee SS, Choung SY, Kang SS, Choi YJ (2016) Preparation and optimisation of liposome-in-alginate beads containing oyster hydrolysate for sustained release. Int J Food Sci Technol 51:2209–2216
Xue X, Zhou Z, Peng B et al (2015) Review on nanomaterials synthesized by vapor transport method: growth and their related applications. RSC Adv 5:79249–79263. https://doi.org/10.1039/c5ra13349a
Yan R, Zhang L, Yang X, Wen C, Zhou Y (2016) Bioavailability evaluation of zinc-bearing palygorskite as a zinc source for broiler chickens. Appl Clay Sci 119:155–160
Yantasee W, Warner CL, Sangvanich T et al (2007) Removal of heavy metals from aqueous systems with thiol functionalized superparamagnetic nanoparticles. Environ Sci Technol 41:5114–5119. https://doi.org/10.1021/es0705238
Yao MF, Li B, Ye HW, Huang WH, Luo QX, Xiao H, McClements DJ, Li LJ (2018) Enhanced viability of probiotics (Pediococcus pentosaceus Li05) by encapsulation in microgels doped with inorganic nanoparticles. Food Hydrocoll 83:246–252
Yerramilli M, Longmore N, Ghosh S (2018) Stability and bioavailability of curcumin in mixed sodium caseinate and pea protein isolate nanoemulsions. J Am Oil Chem Soc 95:1013–1026. https://doi.org/10.1002/aocs.12084
Yi J, Li Y, Zhong F, Yokoyama W (2015) Corrigendum to “the physicochemical stability and in vitro bioaccessibility of beta-carotene in oil-in-water sodium caseinate emulsions” [food hydrocoll 35 (March 2014) 19–27]. Food Hydrocoll 51:519. https://doi.org/10.1016/j.foodhyd.2015.05.005
Yin J, Li X, Li D, Yue T, Fang Q, Ni J, Zhou X, Wu G (2009) Dietary supplementation with zinc oxide stimulates ghrelin secretion from stomach of young pigs. J Nutr Biochem 20:783–790
Zadmajid V, Mohammadi C (2017) Dietary thyme essential oil (Thymus vulgaris) changes serum stress markers, enzyme activity, and hematological parameters in gibel carp (Carassius auratus gibelio) exposed to silver nanoparticles. Iran J Fish Sci 16:1063–1084
Zanella D, Bossi E, Gornati R, Bastos C, Faria N, Bernardini G (2017) Iron oxide nanoparticles can cross plasma membranes. Sci Rep 7:11413
Zema P, Pilosof AMR (2018) On the binding of folic acid to food proteins performing as vitamin micro/nanocarriers. Food Hydrocoll 79:509–517. https://doi.org/10.1016/j.foodhyd.2018.01.021
Zempleni J, Aguilar-Lozano A, Sadri M, Sukreet S, Manca S, Wu D, Zhou F, Mutai E (2017) Biological activities of extracellular vesicles and their cargos from bovine and human milk in humans and implications for infants. J Nutr 147:3–10
Zhang J, Wang D, Wu Y et al (2018) Lipid–polymer hybrid nanoparticles for oral delivery of Tartary buckwheat flavonoids. J Agric Food Chem 66:4923–4932. https://doi.org/10.1021/acs.jafc.8b00714
Zheng B, Peng S, Zhang X, McClements DJ (2018) Impact of delivery system type on curcumin bioaccessibility: comparison of curcumin-loaded nanoemulsions with commercial curcumin supplements. J Agric Food Chem 66:10816–10826. https://doi.org/10.1021/acs.jafc.8b03174
Zhou XX, Wang YB, Gu Q, Li WF (2009) Effects of different dietary selenium sources (selenium nanoparticle and selenomethionine) on growth performance, muscle composition and glutathione peroxidase enzyme activity of crucian carp (Carassius auratus gibelio). Aquaculture 291:78–81
Zhou W, Kornegay ET, Lindermann MD, Swinkels JW, Welten MK, Wong EA (2014) Stimulation of growth by intravenous injection of copper in weanling pigs. J Anim Sci 72:2395–2403
Download references
Author information
Authors and affiliations.
Department of Dietetics and Applied Nutrition, Amity Institute of Applied Sciences (AIAS), Amity University, Kolkata, West Bengal, India
Joyeta Ghosh
Department of Dietetics and Nutrition, NSHM Knowledge Campus, Kolkata, West Bengal, India
Sudrita Roy Choudhury & Khushboo Singh
Faculty of Medical Sciences, School of Pharmacy, University of the West Indies, St. Augustine, West Indies, Trinidad and Tobago
Madan Mohan Gupta
Institute of Pharmacy, Assam Don Bosco University, Sonapur, Assam, India
Deepak Sharma
You can also search for this author in PubMed Google Scholar
Editor information
Editors and affiliations.
Postgraduate Department of Chemistry, Government Postgraduate College Rajouri, Rajouri, Jammu and Kashmir, India
Shakeel Ahmed
Rights and permissions
Reprints and permissions
Copyright information
© 2024 The Author(s), under exclusive license to Springer Nature Singapore Pte Ltd.
About this chapter
Ghosh, J., Choudhury, S.R., Singh, K., Gupta, M.M., Sharma, D. (2024). Biobased Nanomaterials in Nutraceuticals. In: Ahmed, S. (eds) Biobased Nanomaterials. Springer, Singapore. https://doi.org/10.1007/978-981-97-0542-9_13
Download citation
DOI : https://doi.org/10.1007/978-981-97-0542-9_13
Published : 14 May 2024
Publisher Name : Springer, Singapore
Print ISBN : 978-981-97-0541-2
Online ISBN : 978-981-97-0542-9
eBook Packages : Biomedical and Life Sciences Biomedical and Life Sciences (R0)
Share this chapter
Anyone you share the following link with will be able to read this content:
Sorry, a shareable link is not currently available for this article.
Provided by the Springer Nature SharedIt content-sharing initiative
- Publish with us
Policies and ethics
- Find a journal
- Track your research
- Help & FAQ
Bio-based and biodegradable plastics : facts and figures : focus on food packaging in the Netherlands
- BBP Biorefinery & Sustainable Value Chains
- BBP Sustainable Chemistry & Technology
- Biobased Products
Research output : Book/Report › Report › Professional
Publication series
- bioplastics
- food packaging
- packaging materials
- biodegradation
- biobased materials
- biobased economy
- sustainability
This output contributes to the following UN Sustainable Development Goals (SDGs)
Access to Document
- 10.18174/408350
Files and links
- Download PDF
Fingerprint
- Plastics Material Science 100%
T1 - Bio-based and biodegradable plastics : facts and figures : focus on food packaging in the Netherlands
AU - van den Oever, Martien
AU - Molenveld, Karin
AU - van der Zee, Maarten
AU - Bos, Harriëtte
N2 - This report presents an overview of facts and figures regarding bio-based and/or biodegradable plastics, in particular for packaging applications.
AB - This report presents an overview of facts and figures regarding bio-based and/or biodegradable plastics, in particular for packaging applications.
KW - bioplastics
KW - food packaging
KW - packaging materials
KW - biodegradation
KW - biobased materials
KW - biobased economy
KW - sustainability
KW - voedselverpakking
KW - verpakkingsmaterialen
KW - biodegradatie
KW - materialen uit biologische grondstoffen
KW - duurzaamheid (sustainability)
UR - https://edepot.wur.nl/408350
U2 - 10.18174/408350
DO - 10.18174/408350
M3 - Report
T3 - Wageningen Food & Biobased Research
BT - Bio-based and biodegradable plastics : facts and figures : focus on food packaging in the Netherlands
PB - Wageningen Food & Biobased Research
CY - Wageningen
[Databases of the chemical composition of foods in the era of digital nutrition science]
Affiliation.
- 1 Federal Research Centre of Nutrition, Biotechnology and Food Safety, 109240, Moscow, Russian Federation.
- PMID: 32986334
- DOI: 10.24411/0042-8833-2020-10058
By studying the chemical composition of foods, expanding the list of data on the content of nutrients, including minor biologically active substances, in the era of digital nutrition science, it became possible to create relevant systematic databases of the chemical composition of foods and rations in general. They allow us to solve various problems of modern society from the point of view of nutrition science. This review aim to analyze and generalize modern approaches to the formation and updating of databases of the chemical composition of food products from the standpoint of digital nutrition science. Results . This review considers the main provisions regarding creation of databases, directions for the development of food chemistry, discusses existing international programs for collecting and compiling data. The methods of systematizing data on the qualitative composition and content of biologically active and minor substances in products, as well as the problems associated with the development and metrological certification of highly selective highly sensitive analytical methods necessary to obtain reliable and reproducible data are considered. Conclusion . The development of digital nutrition science significantly increases the availability and quality of information on the chemical composition of foods, and allows it to be updated quickly. Further improvement of the quality of the data presented in the tables of chemical composition is associated with the establishment of stability and relationships between micro- and macro-components, their influence on the safety, stability of the chemical structure, the influence of the physic-chemical characteristics of the matrix on nutritive value of foods, determination of the content of specific minor components, development of relevant regulatory documents.
Keywords: databases of the chemical composition of food products; digital nutrition science.
Copyright© GEOTAR-Media Publishing Group.
Publication types
- Databases, Chemical*
- Food Analysis*
- Nutritional Sciences*
- Nutritive Value*
Grants and funding
- The research was carried out at the expense of the subsidy for the implementation of the state task
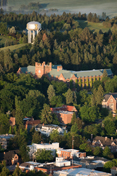
Virtual Tour
Experience University of Idaho with a virtual tour. Explore now
- Discover a Career
- Find a Major
- Experience U of I Life
More Resources
- Admitted Students
- International Students
Take Action
- Find Financial Aid
- View Deadlines
- Find Your Rep
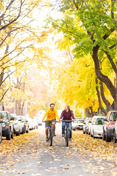
Helping to ensure U of I is a safe and engaging place for students to learn and be successful. Read about Title IX.
Get Involved
- Clubs & Volunteer Opportunities
- Recreation and Wellbeing
- Student Government
- Student Sustainability Cooperative
- Academic Assistance
- Safety & Security
- Career Services
- Health & Wellness Services
- Register for Classes
- Dates & Deadlines
- Financial Aid
- Sustainable Solutions
- U of I Library
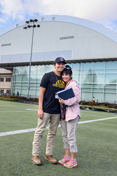
- Upcoming Events
Review the events calendar.
Stay Connected
- Vandal Family Newsletter
- Here We Have Idaho Magazine
- Living on Campus
- Campus Safety
- About Moscow
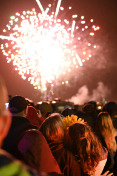
The largest Vandal Family reunion of the year. Check dates.
Benefits and Services
- Vandal Voyagers Program
- Vandal License Plate
- Submit Class Notes
- Make a Gift
- View Events
- Alumni Chapters
- University Magazine
- Alumni Newsletter
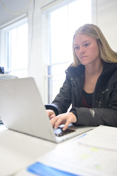
U of I's web-based retention and advising tool provides an efficient way to guide and support students on their road to graduation. Login to VandalStar.
Common Tools
- Administrative Procedures Manual (APM)
- Class Schedule
- OIT Tech Support
- Academic Dates & Deadlines
- U of I Retirees Association
- Faculty Senate
- Staff Council
Food Technology Center
- request product testing
- Take a tour
- request nutrition facts
Mailing Address: University of Idaho 1908 E. Chicago Street Caldwell, ID 83605
Phone: 208-795-5331
Email: [email protected]
Web: uidaho.edu/cals/food-technology
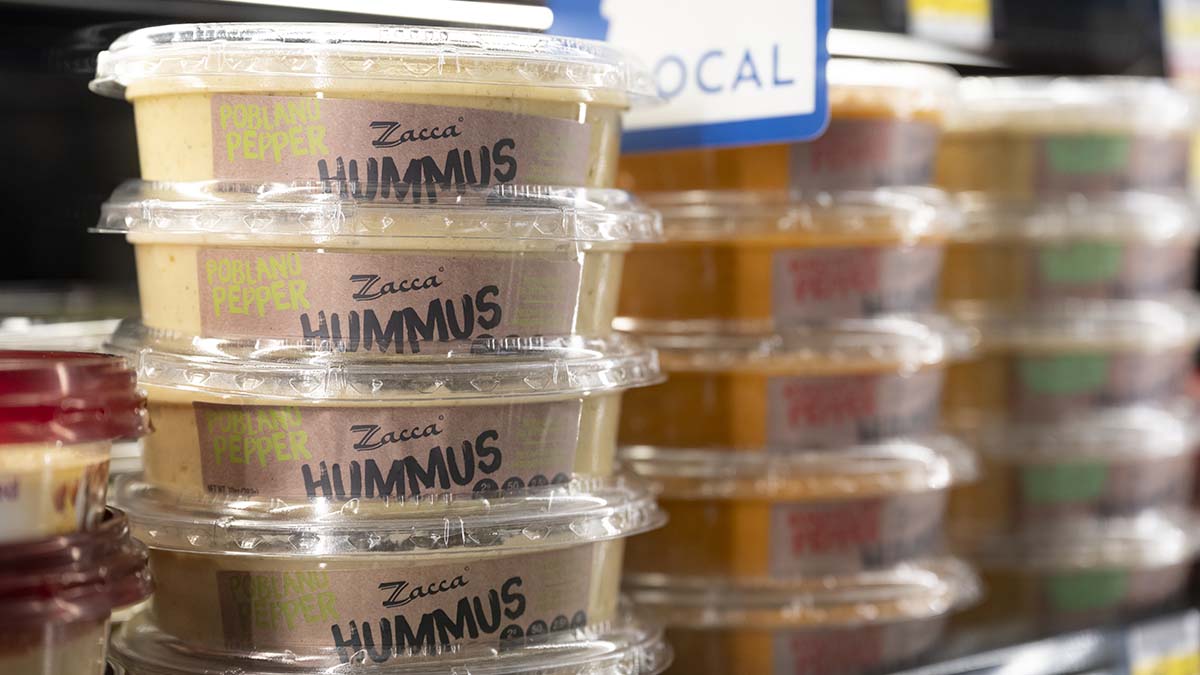
Business Builder
U of I Food Technology Center supports aspiring entrepreneurs who make, market and sell packaged food products
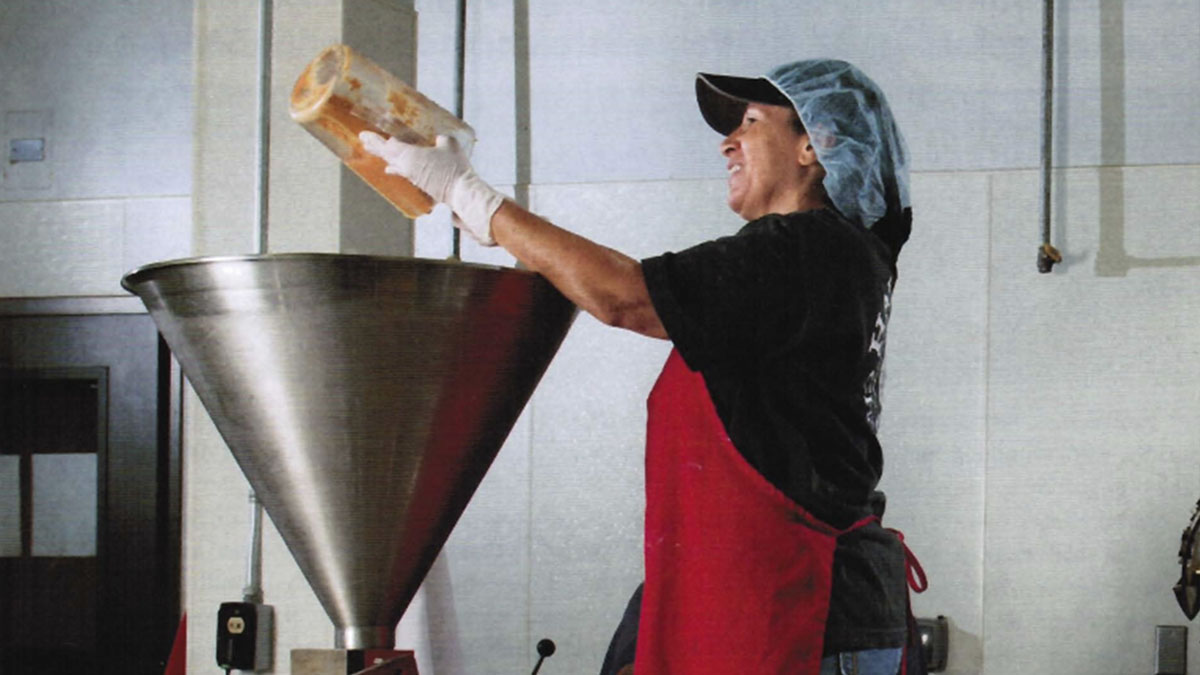
The University of Idaho Food Technology Center offers processing and educational assistance to aspiring food entrepreneurs that wish to produce packaged food products for resale. In addition to the technical support through product and process development, the Food Technology Center includes a commercial kitchen/processing facility, with specialized processing and packaging equipment, where small companies can come get licensed to produce their own packaged food products.
The Food Technology Center also has a pilot plant for larger scale/corporate process testing as well as GLP (Good Laboratory Practices) processing for scientific research.
The Food Technology Center and the U of I Caldwell Campus have the potential and/or resources to serve a variety of functions including:
- Processing and packaging
- Product and process development
- Analytical testing
- Label and packaging development
- Production employee training
- Warehousing services and food storage
Register today Developing a Food Product Idea — Getting started in a packaged food product business In-Person class on Friday, April 26 (8:30 a.m. to 4:30 p.m.) — Fee $125 Gain invaluable insights Regulatory requirements Food safety systems Processing options Marketing strategies Business plans Providing insights into any additional questions you might want to explore
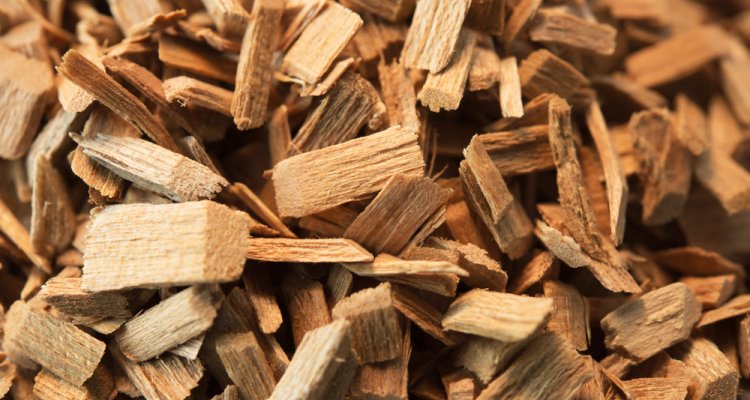
Call for partners | Application and separation of wood vinegar -First the sour then the sweet
Pyrolysis can be used to utilize and valorise lignocellulosic biomass for example residue streams from agriculture and forestry. The primary product of rapid pyrolysis is pyrolysis oil. The by-product from the water removal process is the aqueous condensate that contains a lot of oxygenates such as acetic acid, formic acids, alcohols and phenolics, called wood vinegar. A good application for this stream has not been found yet, while it is important for the economy and circularity of the process.
The TKI project will analyse the composition of wood vinegar and focus on the application of the whole wood vinegar mixture as such and on the isolation of certain valuable compounds. Applications as such may be the use of wood vinegar is a biostimulant in crop production and as a carbon and energy source for denitrification and biological phosphate removal in biological wastewater treatment plants.
The mixture can be used to produce bioplastic (PHA) and to add to manure storage to prevent methane and ammonia emission (by a pH decrease; afterwards the added compounds can be turned into biogas in a biodigester). Isolated acetic acid can be used as a cleaning agent, as a deicer, as a raw material to produce caproic acid and isolated glycolaldehyde can be used to produce glycolic acid (cosmetics, medicines, health care) and polyester (polyglycolic acid). Application research will be carried out and separation processes will be developed to isolate certain compounds.
Already two pyrolysis companies are involved. We need parties that can use the wood vinegar: suppliers and users of biostimulants, owners of wastewater treatment plants, PHA producers seeking for substrates, green herbicide producers. (Representatives of) dairy farmers. Acetic acid users; companies in green cleaning agents, deicers. Polyester producers that would like to produce biobased polyglycolic acid, chemical companies that would like to produce biobased glycolic acids. Companies that supply separation technologies (e.g. membrane modules). We have the intention to start a TKI project on this subject and ask parties to contribute with cash and in kind.
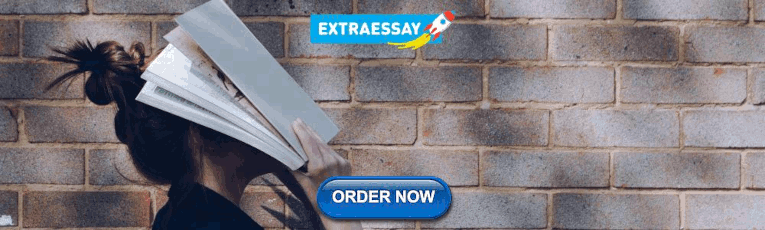
IMAGES
VIDEO
COMMENTS
Research programmes. Wageningen Food & Biobased Research, with our clients and partners, can create economical viable and sustainable solutions to contribute to supplying a rapidly growing world population with healthy, delicious, sustainably produced food and high quality materials, chemicals and fuels made from biomass.
Wageningen Food & Biobased Research develops insights and technologies that support companies, governments and other research institutes in creating innovative solutions for a healthier, more sustainable and prosperous world. We partner in the creation and production of healthy and tasty foods, of truly-sustainable food chains, and in ...
Wageningen Food & Biobased Research helps governments develop well-considered, hands-on strategies to improve fresh logistics, education and (post-harvest) technology in their countries - thereby addressing the entire agrologistics system. "This substantially improves food quality and safety in each country, boosting local
Dive into the research topics of 'Food & biobased research : Healthy and sustainable choices : now and in the future'. Together they form a unique fingerprint. Food Product Food Science 100%
Approaches and limitations for incorporating biomass. There are three research streams that explore the use of biomass for industrial and societal impact (Fig. 1, left): (1) extraction and ...
Fingerprint. Dive into the research topics where Wageningen Food & Biobased Research is active. These topic labels come from the works of this organisation's members. Together they form a unique fingerprint. Fruit Food Science. 100%. Pea Food Science. 84%. Diet Food Science.
(Report / Wageningen Food & Biobased Research; no. 2545) Research output: Book/Report › Report › Professional. Open Access. Carbon Storage 100%. Biogenic Carbon 100%. Global Warming Potential 78%. Potential Benefit 64%. Carbon Dioxide 64%. Biobased grondstoffen voor hoogbouw : geïndustrialiseerde modulaire en lage emissie hoogbouw in de G4
In previous works, a recompilation of the latest research and development on biobased packaging materials and composites form agri-food waste and byproduct was conducted, with a special focus on materials properties and processing technologies for greener production [55,61,62]. Thus, this review gathers the advances reported in the last decade ...
Based on the technical report published by Wageningen Food & Biobased Research in 2017 (van den Oever et al., 2017), it has been shown that the bio-based and biodegradable plastics are currently more expensive than fossil-based plastics on weight basis (Tables 2, 3).However, specific material properties can allow costs reductions in the use or end-of-life phase.
WFBR (Wageningen Food and Biobased Research, one of the research institutes of Wageningen Research, part of WUR.) employs a CAVE-like projection room, where eight beamers can project images onto the walls of a normal room (Figure 4 a). In addition to the visual projections, sounds can be played, and an odour dispersing unit is installed as well.
Wageningen Food & Biobased Research is your trusted partner in addressing consumer behaviour challenges to stimulate healthy and sustainable product and dietary choices. We apply a variety of innovative methods to study product, person, behaviour and context aspects in the lab, in real-life settings, or online to get an integrated approach ...
For 3D printing of biobased products or foods, the following techniques are well-suited: 1) ... Meeting this food security challenge needs to be part and parcel of tackling equally big strategic issues for food research, such as the ageing demographics with a growing population of immunocompromised persons, and ring consumer demand for clean ...
WFBR (Wageningen Food and Biobased Research, one of the research institutes of Wageningen Research, part of WUR.) employs a CAVE-like projection room, where eight beamers can project images onto the walls of a normal room (Figure 4a). In addition to the visual projections, sounds can be played, and an odour dispersing unit is installed as well.
A direct relationship between foods and health has led to various scientific studies to find the significance of foods or food ingredients on specific functions in the body. The term functional food refers to food with specific beneficial functions, is first coined in Japan. The present paper reviews the factors that have driven the functional food development, various definitions proposed by ...
This system categorizes all foods and food products into 4 groups according to the extent and purpose of the industrial processing they undergo (5, 8). In contrast to plant-based meat alternatives and plant-based milks, their animal-based counterparts (beef and cow milk) are classified as unprocessed or minimally processed foods (group 1).
Wageningen Food & Biobased Research evaluates the health benefits of foods from lead finding (screening to identify promising compounds) and preclinical research (in vitro and in vivo), all the way up to human clinical trials. Our ultimate aim is to provide evidence-based information supporting optimal health for all throughout the life span.
Langelaan, manager of the Food Technology research group of Food & Biobased Research, refers to a method in which the use of supercritical CO 2 is combined with extrusion technology: "Tests have shown that this innovative continuous extraction process is less time-consuming, requires less energy and runs at lower costs."
Developing biobased nanotechnology has demonstrated considerable potential for use in functional foods and nutraceuticals to enhance human health. Currently, some of the most important biopolymers and extractives, such as sustainable feedstock, are cellulose, hemicellulose, lignin, and chitosan.
Since its introduction in 1997, the purpose of Food Microbiology: Fundamentals and Frontiers has been to serve as an advanced reference that explores the breadth and depth of food microbiology. Thoroughly updated, the new Fifth Edition adds coverage of the ever-expanding tool chest of new and extraordinary molecular methods to address many of the roles that microorganisms play in the ...
Wageningen Food & Biobased Research. Wageningen Food & Biobased Research, with our clients and partners, can create economical viable and sustainable solutions to contribute to supplying a rapidly growing world population with healthy, delicious, sustainably produced food and high quality materials, chemicals and fuels made from biomass.
PB - Wageningen Food & Biobased Research. CY - Wageningen. ER - van den Oever M, Molenveld K, van der Zee M, Bos H. Bio-based and biodegradable plastics : facts and figures : focus on food packaging in the Netherlands. Wageningen: Wageningen Food & Biobased Research, 2017. 65 p. (Wageningen Food & Biobased Research; 1722). doi: 10.18174/408350
Abstract. By studying the chemical composition of foods, expanding the list of data on the content of nutrients, including minor biologically active substances, in the era of digital nutrition science, it became possible to create relevant systematic databases of the chemical composition of foods and rations in general.
Wageningen Food & Biobased Research is involved in research and development of biobased materials and products. Examples of materials and products we are working on include bioplastics for packaging, casings for consumer electronics, textiles and parts for the automotive industry. We also work on inks, coatings, paper, cardboard, construction materials and biomedical materials.
The Food Technology Center and the U of I Caldwell Campus have the potential and/or resources to serve a variety of functions including: Processing and packaging. Product and process development. Analytical testing. Label and packaging development. Production employee training. Warehousing services and food storage.
I am trained as a chemical engineer and have been working at Wageningen Food and Biobased Research for over 25 years on topics related to biobased and biodegradable polymers. Currently I am programme manager Renewable Plastics. Within this programme our vision is that plastics need to be produced from renewable resources, are recyclable and do ...
Ambitious research being led by scientists at Teesside University is helping to create a circular economy for biomanufacturing industries which rely on fermentation to make their products. Members of the Teesside University Fermochar team, Dr Omar Aboelazayem (left) and Professor David Hughes (right). Teesside University academics are working ...
The synthesis of conventional plastics has increased tremendously in the last decades due to rapid industrialization, population growth, and advancement in the use of modern technologies. However, overuse of these fossil fuel-based plastics has resulted in serious environmental and health hazards by causing pollution, global warming, etc. Therefore, the use of microalgae as a feedstock is a ...
Pyrolysis can be used to utilize and valorise lignocellulosic biomass for example residue streams from agriculture and forestry. The primary product of rapid pyrolysis is pyrolysis oil. The by-product from the water removal process is the aqueous condensate that contains a lot of oxygenates such as acetic acid, formic acids, alcohols and phenolics, called wood vinegar. A good application for ...