The Study of Genetics: Importance for Society Essay
Scientific progress does not stand still but instead constantly stimulates the development of social thought. Thanks to the products of scientific progress, people already use dozens of electronic devices and technological solutions on a daily basis to optimize life. One of the fundamental areas of this development is genetic research. Thus, the study of genetics makes it possible to solve pressing issues related to medicine, forensics, and security. This essay aims to discuss the public importance of supporting genetic research.
With the advances of the genetic sciences, medical progress has reached severe results. A key advantage of genetics for the clinical environment is the real possibility of manipulating the genetic code of a patient’s DNA in order to edit it. Adjustments in the polynucleotide sequences are intended to solve the problem of hereditary diseases and to defeat the harmful, damaging mutations that degrade the quality of life of millions of people. Through genetic manipulation, humanity is expected to be able to solve cancer, type I diabetes, and even schizophrenia (Vincent & Yaghootkar, 2020; Zhuo et al., 2017). Consequently, the benefit of studying genetics for medicine cannot be denied.
On the other hand, encouraging genetic research optimizes forensic systems. By the current moment, many laboratories are using genetics to identify suspects accurately. If traces of the killer are left at the scene of violent crimes, it is possible to qualitatively study their DNA and, consequently, compare it to that of suspects using PCR and electrophoresis techniques (Sebastiana, 2021). In other words, genetic studies simplify the work of law enforcement. So it should be understood that by the same scheme, it is possible to establish paternity, which simplifies the system of legal proceedings and gives reliable results regarding the relationship between the child and the man. Thus, for forensics and genealogy, the use of genetic methods is critical.
Finally, the use of genetics for public safety is of great importance. It should be understood that, in this context, security implies food security because one day, humanity will come to lose sufficient food resources. In such a case, genetic science makes it possible to create genetically modified products, which have a competitive advantage over natural plant forms. By editing the genome of plants, humans can modify their economic properties and thus increase their viability, sustainability, and fertility. That said, the academic community is unequivocally inclined to consider GMOs as utterly safe as conventional food (Biddle, 2018). To put it another way, the use of genetic science has extremely significant implications for the agricultural industry as well. As a result, it allows for the food security of communities in case natural foods are lost.
Finally, it should be emphasized that the promotion of genetic research is directly linked to the improvement of the quality of life of society. Humanity cannot live by conservative rules and abandon scientific and technological progress achievements: such views have a destructive effect. Consequently, the study of genetics is vital for the sustainability of society. As has been shown in this essay, genetics is fundamentally important for medicine, forensics, genealogy, and the agricultural sector. Indeed, the range of functional benefits of genetics is considerably broader. All of this together leads to the conclusion that the genetic sciences must be sustained and that research in these areas is essential to all of society, both in the short and long term.
Biddle, J. B. (2018). “Antiscience Zealotry”? Values, epistemic risk, and the GMO debate. Philosophy of Science, 85 (3), 360-379.
Sebastiana, M. (2021). PCR – polymerase chain reaction. Biologia Vegetal , 1-6.
Vincent, E. E., & Yaghootkar, H. (2020). Using genetics to decipher the link between type 2 diabetes and cancer: Shared aetiology or downstream consequence? Diabetologia, 63 (9), 1-12.
Zhuo, C., Hou, W., Hu, L., Lin, C., Chen, C., & Lin, X. (2017). Genomic editing of non-coding RNA genes with CRISPR/Cas9 ushers in a potential novel approach to study and treat schizophrenia. Frontiers in Molecular Neuroscience, 10 , 28-35.
- Chicago (A-D)
- Chicago (N-B)
IvyPanda. (2022, August 27). The Study of Genetics: Importance for Society. https://ivypanda.com/essays/the-study-of-genetics-importance-for-society/
"The Study of Genetics: Importance for Society." IvyPanda , 27 Aug. 2022, ivypanda.com/essays/the-study-of-genetics-importance-for-society/.
IvyPanda . (2022) 'The Study of Genetics: Importance for Society'. 27 August.
IvyPanda . 2022. "The Study of Genetics: Importance for Society." August 27, 2022. https://ivypanda.com/essays/the-study-of-genetics-importance-for-society/.
1. IvyPanda . "The Study of Genetics: Importance for Society." August 27, 2022. https://ivypanda.com/essays/the-study-of-genetics-importance-for-society/.
Bibliography
IvyPanda . "The Study of Genetics: Importance for Society." August 27, 2022. https://ivypanda.com/essays/the-study-of-genetics-importance-for-society/.
- Friedrich Nietzsche: Values in ‘the Genealogy of Morals’
- DNA and Genealogy Solving Cold Case Murders: The Modern Technology
- "On the Genealogy of Morality": Nietzsche’s Critique of Modern Values
- Friedrich Nietzsche, the Genealogy of Morals
- "On the Genealogy of Morality" by Friedrich Nietzsche: Passage Analysis
- Mitochondria DNA (mtDNA) in Genealogy
- The S. Freud Reader and F. Nietzsche's Genealogy of Morals Spiritual Revelations
- Modern Technology in DNA and Genealogy Solving Cold Case Murders
- "A Genealogy of Modern Racism" by C. West
- The Controversy of Darwin’s Theory
- Resolving Genetic Issues or Playing With Mother Nature?
- The Development of the Neural System and Genetic Program
- Genetic Disorder: “A Genetic Link to Anorexia”
- Genetic Enhancement: Ethical Aspect
- Genetic Modification and Implicit Bias Against People With Disabilities
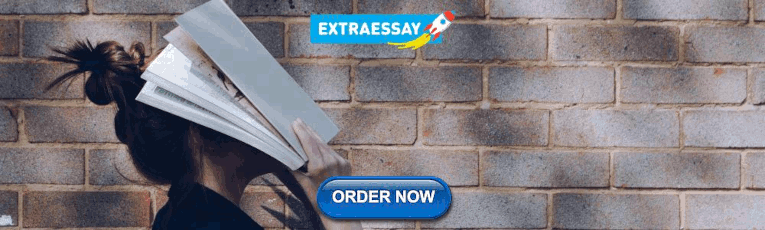
What are the benefits of genetic testing? Experts explain
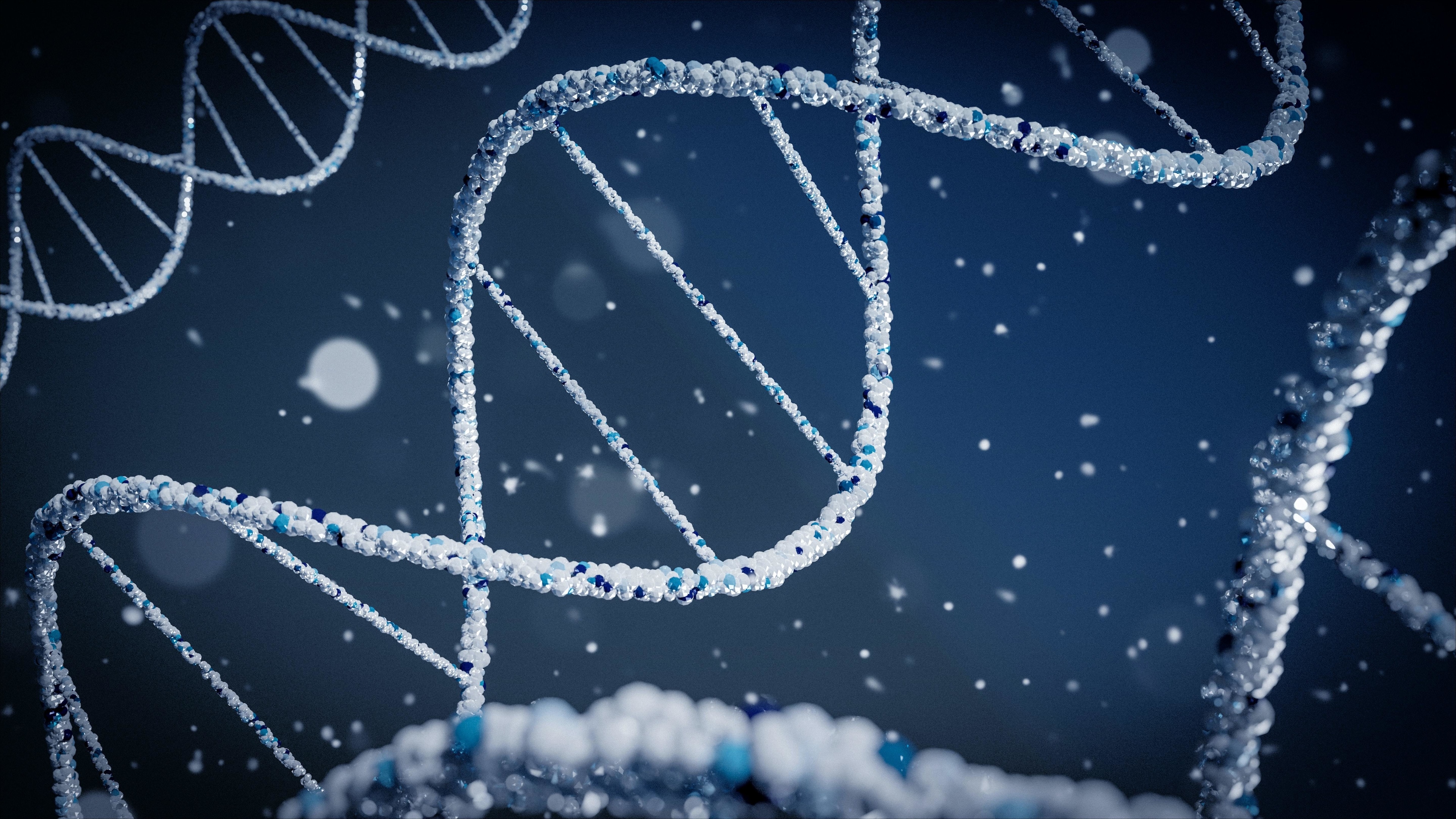
'Precision medicine looks at the interaction of a person's genes, environment and lifestyle, and its contribution to disease.' Image: UNSPLASH/Braňo
.chakra .wef-1c7l3mo{-webkit-transition:all 0.15s ease-out;transition:all 0.15s ease-out;cursor:pointer;-webkit-text-decoration:none;text-decoration:none;outline:none;color:inherit;}.chakra .wef-1c7l3mo:hover,.chakra .wef-1c7l3mo[data-hover]{-webkit-text-decoration:underline;text-decoration:underline;}.chakra .wef-1c7l3mo:focus,.chakra .wef-1c7l3mo[data-focus]{box-shadow:0 0 0 3px rgba(168,203,251,0.5);} Colette Gallagher
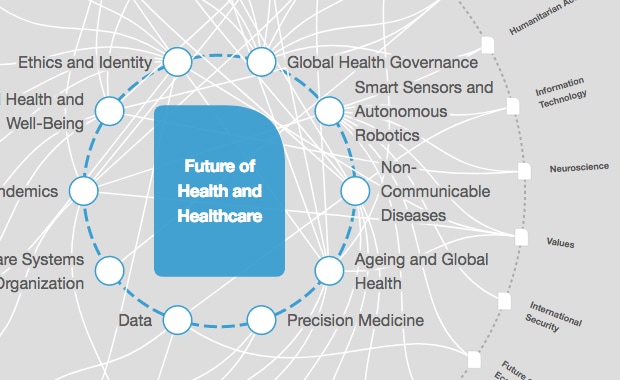
.chakra .wef-9dduvl{margin-top:16px;margin-bottom:16px;line-height:1.388;font-size:1.25rem;}@media screen and (min-width:56.5rem){.chakra .wef-9dduvl{font-size:1.125rem;}} Explore and monitor how .chakra .wef-15eoq1r{margin-top:16px;margin-bottom:16px;line-height:1.388;font-size:1.25rem;color:#F7DB5E;}@media screen and (min-width:56.5rem){.chakra .wef-15eoq1r{font-size:1.125rem;}} Health and Healthcare is affecting economies, industries and global issues
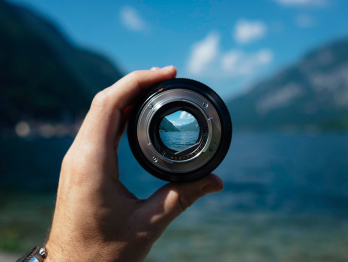
.chakra .wef-1nk5u5d{margin-top:16px;margin-bottom:16px;line-height:1.388;color:#2846F8;font-size:1.25rem;}@media screen and (min-width:56.5rem){.chakra .wef-1nk5u5d{font-size:1.125rem;}} Get involved with our crowdsourced digital platform to deliver impact at scale
Stay up to date:, health and healthcare.
- Genomics experts have released a paper entitled 'The Economic Analysis of the Value of Genetic Testing', containing contributions from across the globe.
- This paper highlights progress in the fields of rare disease, cancer, population health and carries screening.
- Below, the benefits of genetic testing as a preventative healthcare measure are outlined.
Genomics experts worldwide released a paper highlighting advances in rare disease, cancer, population health and carrier screening at the 10th Annual Individualizing Medicine Conference on Saturday, Oct. 9.
"The use cases we explored in the paper, 'The Economic Analysis of the Value of Genetic Testing' are only the tip of the iceberg in the realm of precision medicine," says co-author Konstantinos Lazaridis, M.D., the Carlson and Nelson Endowed Executive Director for Mayo Clinic's Center for Individualized Medicine . "We found with the development of more effective sequencing methods, the prospect of truly moving genomics to the clinic for a wide variety of uses will become a reality."
Precision medicine looks at the interaction of a person's genes, environment and lifestyle, and its contribution to disease. It aims to find the right treatment for the right person at the right time for disease treatment and prevention.
Genetic testing offers many benefits, such as:
- Diagnosing and assessing the severity of disease.
- Predicting a patient's risk for developing a type of disease.
- Revealing the likelihood of passing on a disease to children.
Today, sequencing costs may be as low as $1,000 and results take only days or weeks instead of years to deliver to patients. When the human genome was mapped in 2003, it took 13 years to complete and cost $5.34 billion.
Big data sets are another critical advancement in making genomics more feasible. Advances in computational and statistical methods allow researchers and physicians to use genomic data and find targeted therapies for a patient, according to Cameron Fox, a co-author from the World Economic Forum.
Have you read?
This device can diagnose a genetic disease in 15 minutes, genetic report cards could be the future of healthcare, rare disease testing, diagnosis leads to more cost-effective medical care.
Approximately 300 million people worldwide suffer from a rare disease, with "rare" defined as conditions that affect less than 1 in 2,000 people. Genetic factors cause 80% of these disorders. The quest for a diagnosis averages five years, and genetic testing and diagnosis remains a challenge.
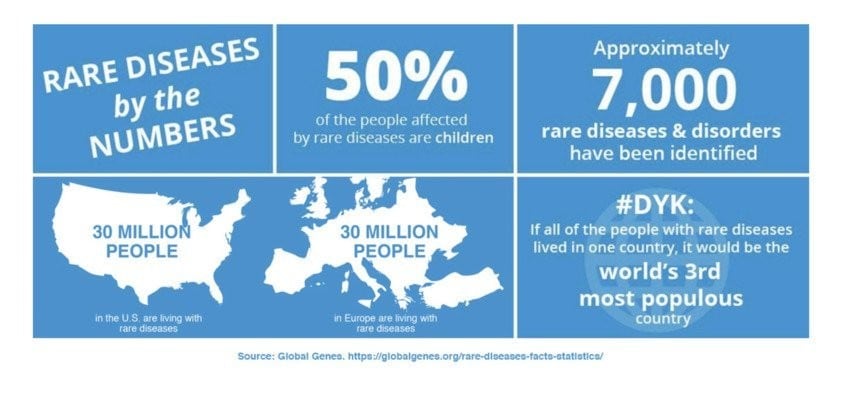
Dr. Lazaridis notes the annual cost of treating rare diseases is many times higher than cancer and other notable common disorders.
"Genomic sequencing has the potential to lead the charge in a new standard of care for rare diseases," says Dr. Lazaridis. "However, rare diseases need to be coded better, and tracked in health care and other systems, so we can achieve faster, better and more cost-effective results."
Broad panel testing for lung cancer
Lung cancer is the second most diagnosed cancer and the leading cause of cancer deaths worldwide. Understanding the various genetic pathways leading to lung cancer has given rise to targeted treatments to attack cancer cells to minimize potential side effects from normal cells and tissue damage.
Testing for these mutations is an essential part of the diagnosis and treatment process. Approximately 40%–50% of lung cancers exhibit a targetable gene mutation. Appropriate, targeted treatment can increase the length of time that a patient lives with cancer and it does not worsen.
Data suggest that a broad panel test for many mutations versus narrow panel testing (fewer genes tested) can be a cost-effective way to determine the correct treatment for a patient, and this is a valuable solution to complex genetic testing. A retrospective review noted in the paper showed that patients with lung cancer who received broad panel testing might have had higher costs, but the overall cost of cancer care was significantly lower. Other studies supported these findings. Also, the cost of adding more genes to broad panels was not significant after a certain point, lending further acceptance to their use.
Liver cancer screening in community-based population health
The population health approach aims to improve the physical and mental health outcomes, and the well-being of people within defined populations to reduce health inequalities.
The authors featured liver cancer cases in China as a model to explain the public health benefit of community-based population health testing. Liver cancer is one of the most common cancer types globally, and it is challenging to treat in its mid to late stages.
China is home to nearly 50% of new liver cancer cases and deaths worldwide. China's disproportionately high death rate is due to approximately 80% of patients diagnosed at the late stage, when treatment options are limited or expensive. Among China's high-risk population, 87 million people are hepatitis B virus carriers, an affliction that significantly elevates their risk of developing liver cancer.
Using liver cancer as a model, Genetron Holdings Ltd. works with the Wuxi Municipal People's Government (Jiangsu Province) to offer early liver cancer screening as a public health benefit to people in the city who are at high risk of developing liver cancer. By raising public awareness, detecting and diagnosing liver cancer will shift to earlier stages, significantly increasing the five-year survival rate and removing costs from the health care system.
Carrier screening for certain disorders
Couples seeking to start a family often seek out carrier screening. This test determines the risk of their offspring being born with a genetic condition, including life-limiting and medically manageable conditions.
Before pregnancy, there is considerable disagreement on what genes or diseases to include for carrier testing (genes screened based on a population or heritage versus the most common disease or syndromes). Also, there is the potential challenge of interpreting genetic variation, especially new variation, in the absence of an affected child.
While ethical dilemmas arise around carrier screening before attempting to become pregnant, offering screening in this context has advantages for a couple considering having children in the future.
Population carrier screening for at-risk communities
In response to the increased risk of certain conditions, some ethnic groups have developed carrier screening programs targeted to conditions present in their population.
Consider these examples:
- The U.S. Amish, Mennonite and Hutterite groups focus on patient education and genetic counseling to identify and treat people with inherited single-gene disorders.
- The American College of Obstetricians and Gynecologists recommends that women of Southeast Asian ancestry be screened for thalassemia. This red blood cell disorder can reduce blood oxygenation, leading to anemia.
- The Orthodox Jewish community created the Dor Yeshorim Program, which determines the "genetic compatibility" of couples interested in marriage with the hopes of preventing debilitating diseases.
The authors note targeted testing can provide an at-risk community with information to guide decision-making, maintain confidentiality, and support mental and emotional well-being.
With all of these advances in rare disease, cancer, population health and carrier screening, there is increasing need to provide timely, equitable access to genomics tools in the health care system.
"We need communities, policymakers, payers, physicians, scientists, genetic counselors, among others to help us speed up the process and move genomics into the clinic to save time, lives and resources," says Fox.
The Moving Genomics to the Clinic Project is led by the World Economic Forum’s Platform for Shaping the Future of Health and Healthcare. The paper was produced by four working groups focusing on cancer, rare disease, population health, and carrier screening in collaboration with several organizations from across the health care ecosystem.
The project aims to explore, design and test incentives to accelerate building the evidence base for clinical efficacy and utility as a way to increase coverage of genetic testing and screening. The World Economic Forum consists of the foremost political, business, cultural and other leaders of society to shape global, regional and industry agendas.
Each year, $3.2 trillion is spent on global healthcare making little or no impact on good health outcomes.
To address this issue, the World Economic Forum created the Global Coalition for Value in Healthcare to accelerate value-based health systems transformation.
This council partners with governments, leading companies, academia, and experts from around the world to co-design and pilot innovative new approaches to person-centered healthcare.
Co-authors are:
Lynsey Chediak, head, Partnerships, Rarebase.
Anne Claussen, vice president, Cancer and Other Serious Illnesses Transformation, CVS Health.
Katherine Dunn, associate adviser, Intermountain Healthcare.
Kirsten Farncombe, scientific associate, Toronto General Hospital Research Institute, University Health Network.
Jason Flanagan, genetic counselor, Sanford Health.
Cameron Fox, World Economic Forum
Panos Kanavos, deputy director, LSE Health.
Raymond Kim, clinician-scientist, Princess Margaret Cancer Centre, University Health Network, Department of Medicine, University of Toronto.
Konstantinos Lazaradis, M.D., Mayo ClinicChristy Moore, program manager, Clinical Genetics, Blue Shield of California.
Jeff Niu, innovation and experience lead, Product and Strategy, Genetron Holdings Ltd.
Maria Raimundo, senior account manager, Beta-I.
Shirisha Reddy, medical director, CVS Health.Caoimhe Vallely-Gilroy, global head, Digital Health and Therapeutics, Merck & Co. Inc.
Bryce Waldman, strategy and business operations, Invitae Corp.
Christina Waters, senior adviser, Congenica Ltd.
Don't miss any update on this topic
Create a free account and access your personalized content collection with our latest publications and analyses.
License and Republishing
World Economic Forum articles may be republished in accordance with the Creative Commons Attribution-NonCommercial-NoDerivatives 4.0 International Public License, and in accordance with our Terms of Use.
The views expressed in this article are those of the author alone and not the World Economic Forum.
Related topics:
The agenda .chakra .wef-n7bacu{margin-top:16px;margin-bottom:16px;line-height:1.388;font-weight:400;} weekly.
A weekly update of the most important issues driving the global agenda
.chakra .wef-1dtnjt5{display:-webkit-box;display:-webkit-flex;display:-ms-flexbox;display:flex;-webkit-align-items:center;-webkit-box-align:center;-ms-flex-align:center;align-items:center;-webkit-flex-wrap:wrap;-ms-flex-wrap:wrap;flex-wrap:wrap;} More on Health and Healthcare .chakra .wef-17xejub{-webkit-flex:1;-ms-flex:1;flex:1;justify-self:stretch;-webkit-align-self:stretch;-ms-flex-item-align:stretch;align-self:stretch;} .chakra .wef-nr1rr4{display:-webkit-inline-box;display:-webkit-inline-flex;display:-ms-inline-flexbox;display:inline-flex;white-space:normal;vertical-align:middle;text-transform:uppercase;font-size:0.75rem;border-radius:0.25rem;font-weight:700;-webkit-align-items:center;-webkit-box-align:center;-ms-flex-align:center;align-items:center;line-height:1.2;-webkit-letter-spacing:1.25px;-moz-letter-spacing:1.25px;-ms-letter-spacing:1.25px;letter-spacing:1.25px;background:none;padding:0px;color:#B3B3B3;-webkit-box-decoration-break:clone;box-decoration-break:clone;-webkit-box-decoration-break:clone;}@media screen and (min-width:37.5rem){.chakra .wef-nr1rr4{font-size:0.875rem;}}@media screen and (min-width:56.5rem){.chakra .wef-nr1rr4{font-size:1rem;}} See all
Scientists have invented a method to break down 'forever chemicals' in our drinking water. Here’s how
Johnny Wood
April 17, 2024
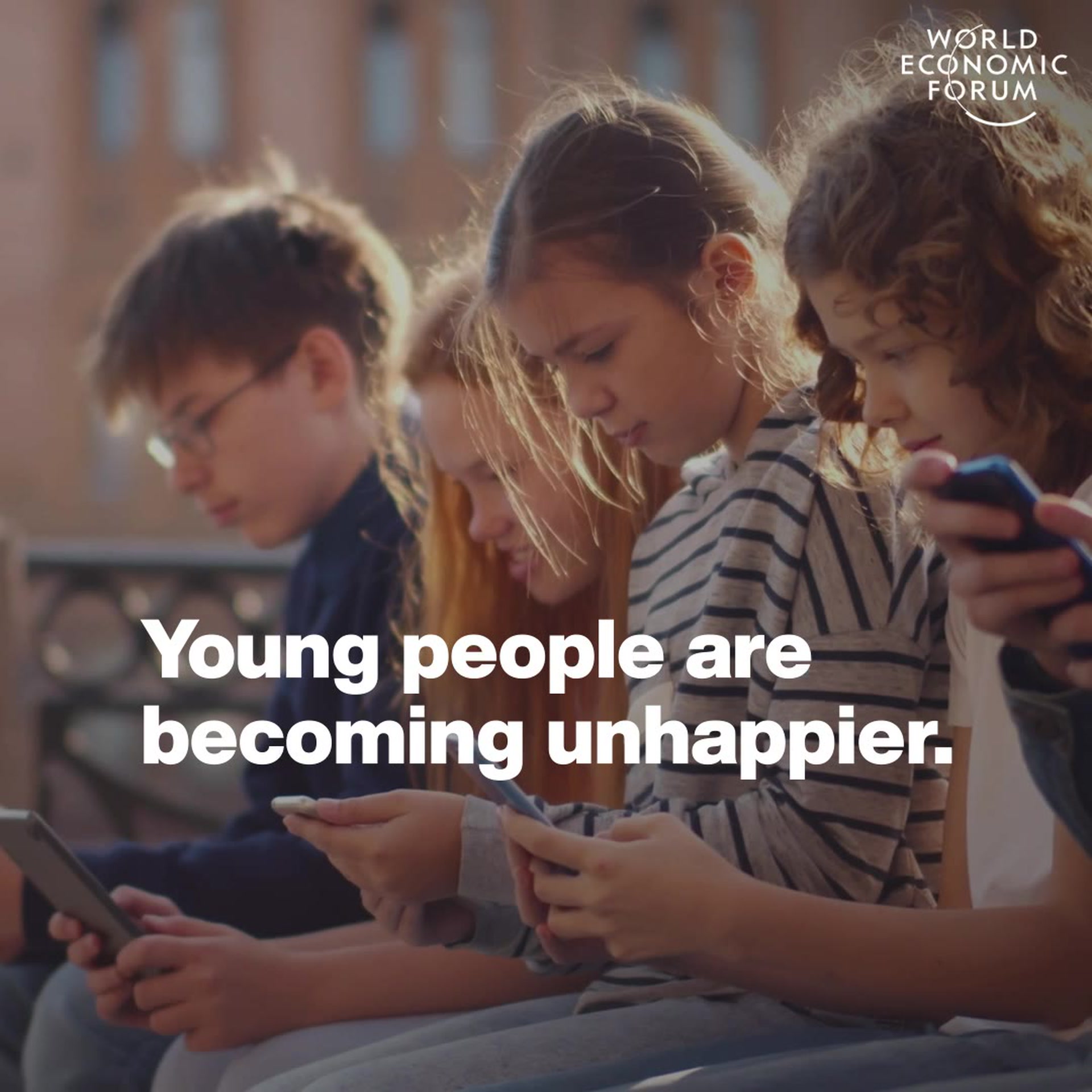
Young people are becoming unhappier, a new report finds
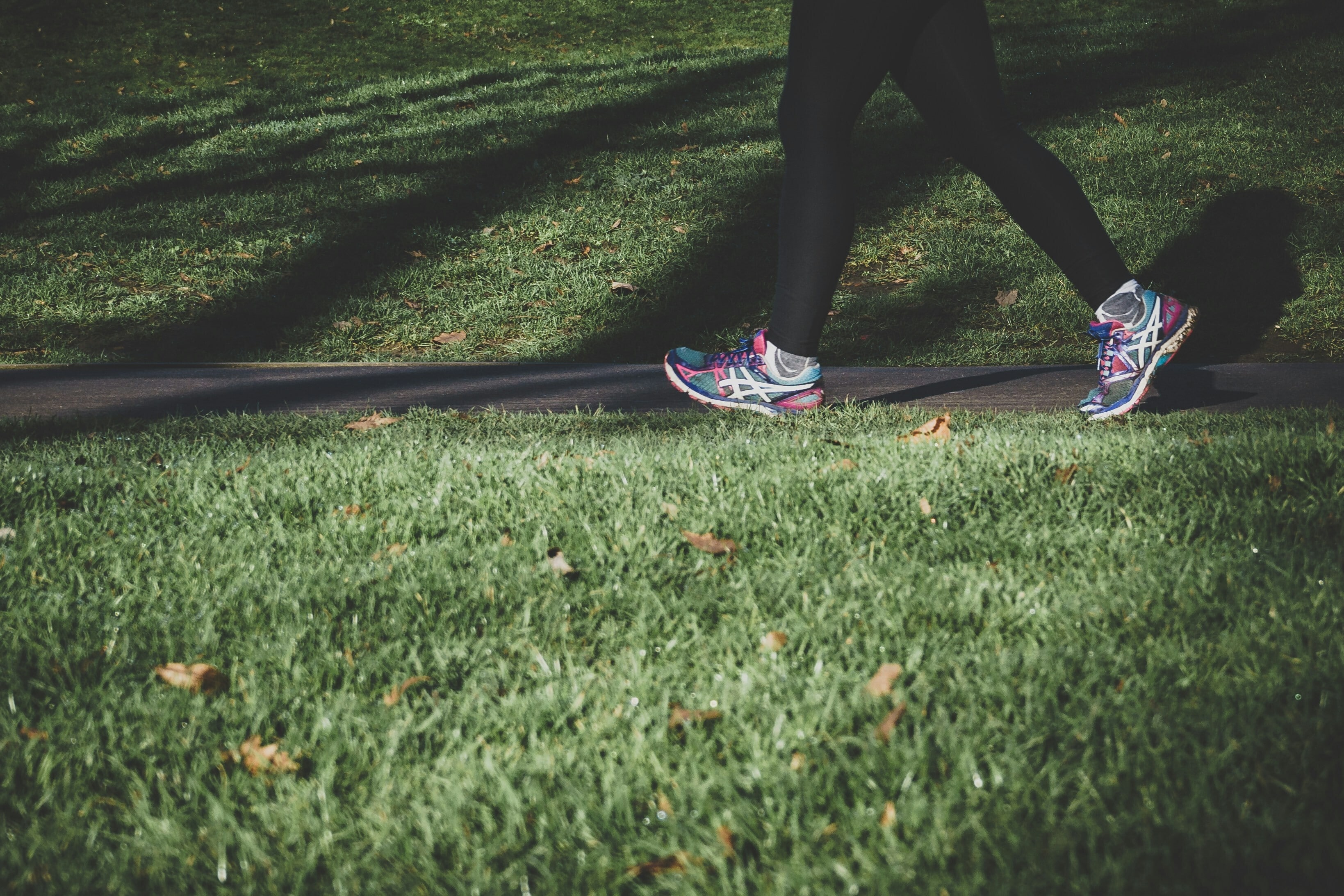
Promoting healthy habit formation is key to improving public health. Here's why
Adrian Gore
April 15, 2024
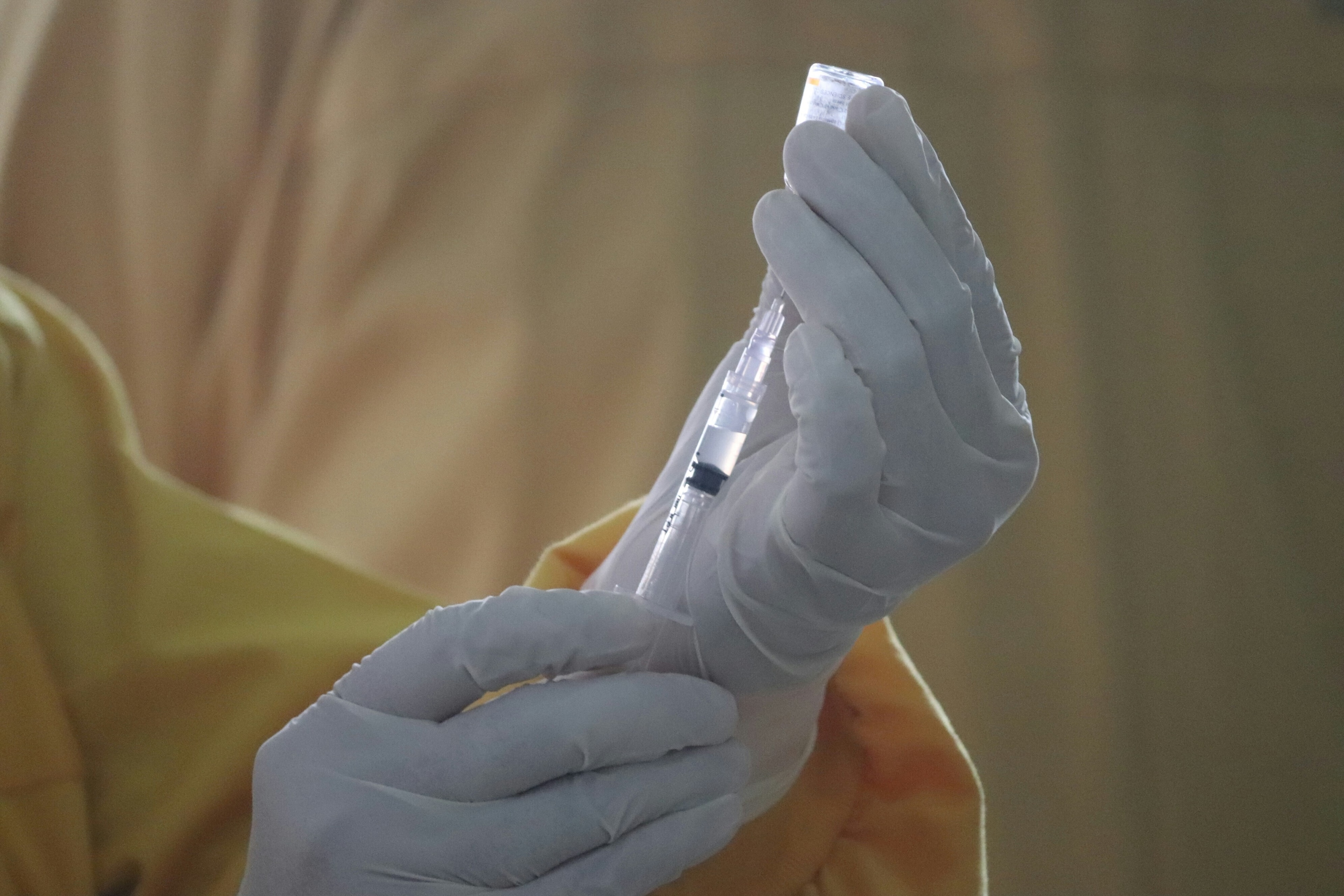
Why stemming the rise of antibiotic resistance will be an historic achievement
Carel du Marchie Sarvaas
April 11, 2024
Medical innovation for an aging world: the view from Hong Kong
April 10, 2024
Kidney disease ‘should be global health priority’, plus other top health stories
Shyam Bishen
- Português Br
- Journalist Pass
Science Saturday: The benefits of genetic testing for healthy people
Colette Gallagher
Share this:
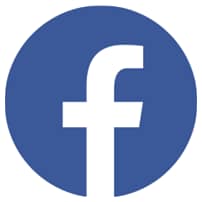
More than 1 in 10 people who had predictive genomic testing ― testing that looks for genetic mutations in otherwise healthy people ― learned that they had a hereditary risk for a health condition and may benefit from preventive care, according to a recent study published in Mayo Clinic Proceedings . This study is the first to assess predictive genetic testing in a clinical setting.
Researchers found nearly 12% of people had findings that were "clinically actionable," meaning they had genes that increased their risk for various types of cancer, cardiovascular disease or a negative reaction to anesthesia. Therefore, they may be candidates for preventive care, such as regular screening, preventive surgery, or receiving or avoiding specific anesthetics.
"About half of those patients did not have a personal or family medical history that would suggest they had this risk, meaning it likely would not otherwise have been identified," says Jennifer L. Anderson, a Mayo Clinic genetic counselor and the study's lead author. "Our findings suggest that many people who are generally healthy may have a genetic risk, and broader adoption of predictive genetic testing could be beneficial in routine medical practice ― ideally including genetic counseling."
Between 2014 and 2019, 301 of 1,281 patients pursued predictive genetic testing at Mayo's Predictive Genomics Clinic, where diagnosis and treatment are individually tailored.
- Overall, 11.6% of patients, or 35 of 301 of patients, had a gene that was associated with a hereditary risk, most commonly the BCHE, BRCA2, CHEK2, LDLR, MUTYH or MYH7 genes. These genes are associated with health issues such as a negative reaction to certain types of anesthesia, breast and colon cancers, high cholesterol, or heart disease.
- Of the 29 patients with hereditary cancer or cardiovascular risks identified, 51.7% of patients, or 15 patients, had no family or medical history of the genetic risk.
- Also, 55.8% of patients, or 168 of 301 patients, were carriers of at least one recessive gene. This means that while their health likely isn't affected, their children's health could be. If both parents carry the same recessive gene and pass it on, the child would have a risk for the genetic condition.
- A gene associated with multifactorial disease, which is a disease caused by multiple genetic and environmental factors, was found in 42.5% of patients, or 128 patients. This gene is just one of a number of aspects, in addition to lifestyle choices and environment that increase the risk of developing a health condition such as Alzheimer's disease.
The number of people who decided to pursue testing at Mayo Clinic increased from 11% in 2014 to 40% in 2019, according to Anderson.
"We have found that patients are increasingly interested in this type of predictive test and want to learn about their genetic risk for diseases, even if they do not have a personal or family history of those conditions," says Anderson. "Genetic counseling is an important part of predictive testing by helping patients understand the results and their options for preventive care."
Read more stories about advances in individualized medicine.
Register to get weekly updates from the Mayo Clinic Center for Individualized Medicine blog .
Join the conversation
For more information on the Mayo Clinic Center for Individualized Medicine , visit Facebook , LinkedIn or Twitter at @MayoClinicCIM
- Team among first to receive COVID-19 vaccine at Mayo Clinic in Rochester Mayo Clinic Q&A podcast: Study finds unique form of immunosuppression caused by brain cancer
Related Articles
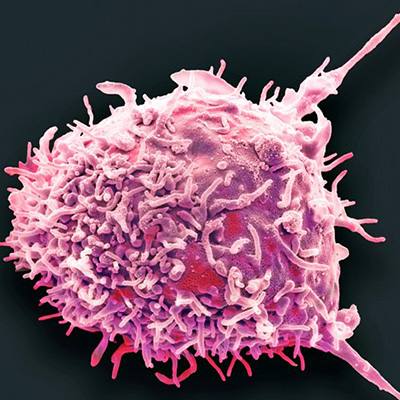

Understanding the Role of Genetic Health Testing
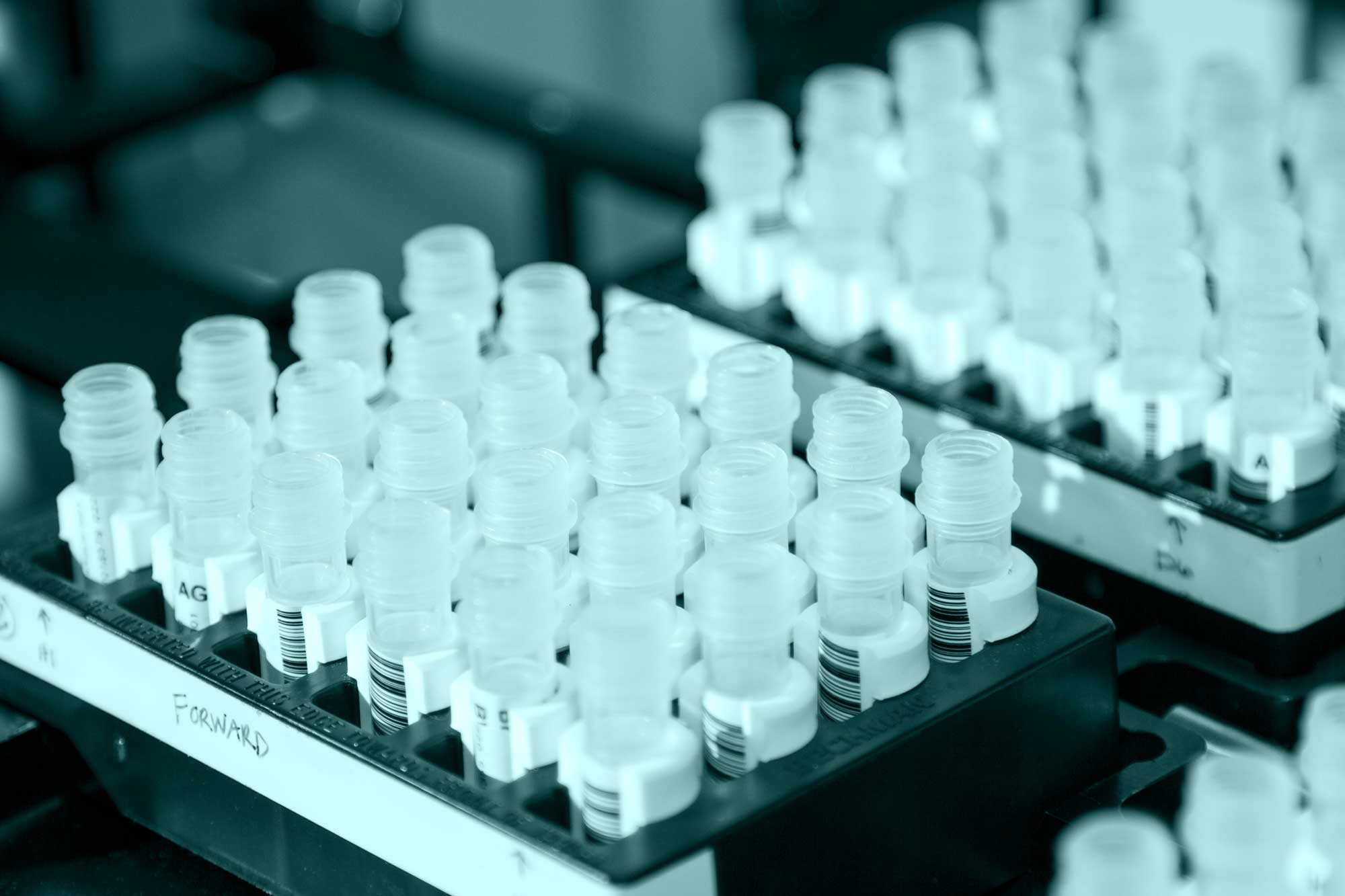
Every cell in your body contains a set of instructions called genes, which help determine how your body works and your individual physical characteristics. You inherit a unique combination of genes from your parents. These genes, together with many other factors, such as your environment and your lifestyle choices, make us unique individuals. Genes influence your height, hair color, aspects of your personality, risk for developing a variety of diseases, and how you will respond to certain medications.
An inherited trait is one that is genetically determined. Most traits are not strictly determined by genes, but rather are influenced by both genes and environment.
Over the past few decades, our understanding of how genes influence our health has increased significantly. Our ability to determine how an individual’s genes may impact a person’s health is especially useful, because that information can be used to help guide disease prevention and treatment strategies.
Genetic Testing
Genetic tests determine variations in genes. They may be used to help guide medical decisions, identify individuals, or provide information about how your body works. Genetic test results may encourage you to be proactive about lifestyle changes that may help manage health risks.
A genetic marker is a DNA sequence with a known physical location on a chromosome. Genetic markers can help link an inherited disease with the responsible gene.
Your doctor may recommend a genetic health test for you if certain health conditions run in your family or if you or a family member has been diagnosed with a condition known to have a genetic marker that may impact treatment decisions. Consumer genetic health testing is available if you are interested in learning about your comparative risk of disease or how you may respond to specific medicines. The test results may encourage you to be proactive about lifestyle changes that may help you manage health risks. For example, if you have an increased genetic risk of developing celiac disease, you might change your diet and exercise habits and ask your healthcare provider to help you develop a plan to watch for early symptoms of disease.
The Role of Confirmatory Testing
Some genetic health-related information is “medically actionable,” meaning medical interventions are available that could reduce your risk of getting a disease or that using this information could influence treatment decisions and how the disease advances. In this case, you should talk to your doctor about your results. Before beginning any strategy, your doctor may want you to test again or confirm your results through another method.
If a test reveals you have an equal or lower risk of developing a specific illness than the general population, you should still receive recommended health screenings such as mammograms and blood tests. Your environment and other lifestyle factors also affect your health. Regular checkups and a healthy lifestyle are very important, regardless of your genetic risk factors. As with any health-related decision, you should work with your healthcare provider to develop a health management strategy that suits your needs.
More Information
For more information about the risks and benefits of genetic health testing, please see the Personalized Medicine Coalition's Consumer’s Guide to Genetic Health Testing .
Related Articles

Will My Insurance Coverage Be Affected By My Genetic Information?
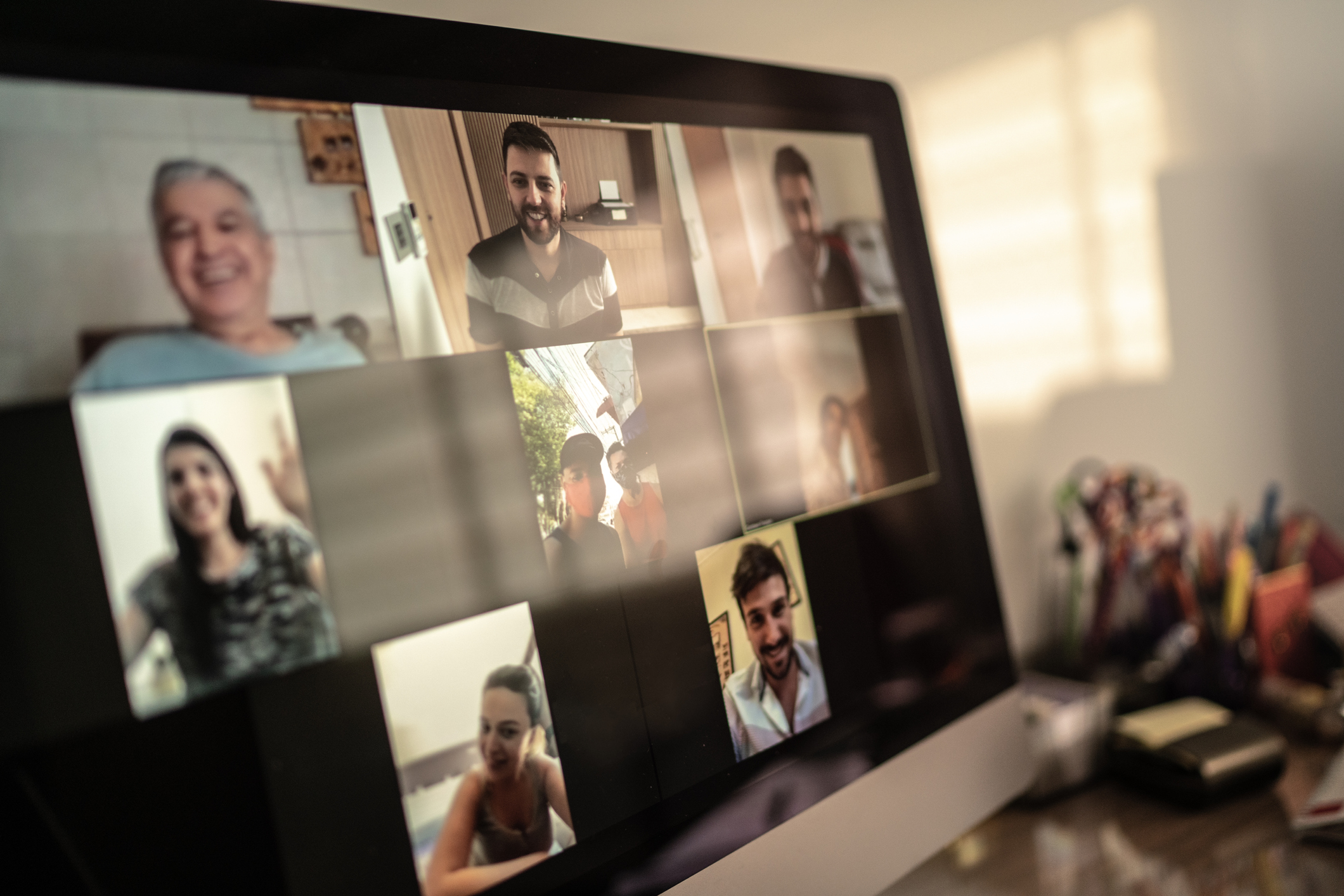
Creating and Understanding a Family Health History
Thank you for visiting nature.com. You are using a browser version with limited support for CSS. To obtain the best experience, we recommend you use a more up to date browser (or turn off compatibility mode in Internet Explorer). In the meantime, to ensure continued support, we are displaying the site without styles and JavaScript.
- View all journals
- Explore content
- About the journal
- Publish with us
- Sign up for alerts
- Published: 24 August 2020
The road ahead in genetics and genomics
- Amy L. McGuire 1 ,
- Stacey Gabriel 2 ,
- Sarah A. Tishkoff ORCID: orcid.org/0000-0002-1339-5959 3 , 4 ,
- Ambroise Wonkam ORCID: orcid.org/0000-0003-1420-9051 5 , 6 ,
- Aravinda Chakravarti ORCID: orcid.org/0000-0002-4264-2285 7 ,
- Eileen E. M. Furlong ORCID: orcid.org/0000-0002-9544-8339 8 ,
- Barbara Treutlein ORCID: orcid.org/0000-0002-3299-5597 9 ,
- Alexander Meissner ORCID: orcid.org/0000-0001-8646-7469 2 , 10 , 11 , 12 ,
- Howard Y. Chang ORCID: orcid.org/0000-0002-9459-4393 13 ,
- Núria López-Bigas ORCID: orcid.org/0000-0003-4925-8988 14 , 15 , 16 ,
- Eran Segal ORCID: orcid.org/0000-0002-6859-1164 17 &
- Jin-Soo Kim ORCID: orcid.org/0000-0003-4847-1306 18
Nature Reviews Genetics volume 21 , pages 581–596 ( 2020 ) Cite this article
80k Accesses
110 Citations
343 Altmetric
Metrics details
- Genetic techniques
In celebration of the 20th anniversary of Nature Reviews Genetics , we asked 12 leading researchers to reflect on the key challenges and opportunities faced by the field of genetics and genomics. Keeping their particular research area in mind, they take stock of the current state of play and emphasize the work that remains to be done over the next few years so that, ultimately, the benefits of genetic and genomic research can be felt by everyone.
The contributors
Amy L. McGuire is the Leon Jaworski Professor of Biomedical Ethics and Director of the Center for Medical Ethics and Health Policy at Baylor College of Medicine. She has received numerous teaching awards at Baylor College of Medicine, was recognized by the Texas Executive Women as a Woman on the Move in 2016 and was invited to give a TedMed talk titled “There is No Genome for the Human Spirit” in 2014. In 2020, she was elected as a Hastings Center Fellow. Her research focuses on ethical and policy issues related to emerging technologies, with a particular focus on genomic research, personalized medicine and the clinical integration of novel neurotechnologies.
Stacey Gabriel is the Senior Director of the Genomics Platform at the Broad Institute since 2012 and has led platform development, execution and operation since its founding. She is Chair of Institute Scientists and serves on the institute’s executive leadership team. She is widely recognized as a leader in genomic technology and project execution. She has led the Broad’s contributions to numerous flagship projects in human genetics, including the International HapMap Project, the 1000 Genomes Project, The Cancer Genome Atlas, the National Heart, Lung, and Blood Institute’s Exome Sequencing Project and the TOPMed programme. She is Principal Investigator of the Broad’s All of Us (AoU) Genomics Center and serves on the AoU Program Steering Committee.
Sarah A. Tishkoff is the David and Lyn Silfen University Associate Professor in Genetics and Biology at the University of Pennsylvania, Philadelphia, USA, and holds appointments in the School of Medicine and the School of Arts and Sciences. She is a member of the US National Academy of Sciences and a recipient of an NIH Pioneer Award, a David and Lucile Packard Career Award, a Burroughs/Wellcome Fund Career Award and an American Society of Human Genetics Curt Stern Award. Her work focuses on genomic variation in Africa, human evolutionary history, the genetic basis of adaptation and phenotypic variation in Africa, and the genetic basis of susceptibility to infectious disease in Africa.
Ambroise Wonkam is Professor of Medical Genetics, Director of GeneMAP (Genetic Medicine of African Populations Research Centre) and Deputy Dean Research in the Faculty of Health Sciences, University of Cape Town, South Africa. He has successfully led numerous NIH- and Wellcome Trust-funded projects over the past decade to investigate clinical variability in sickle cell disease, hearing impairment genetics and the return of individual findings in genetic research in Africa. He won the competitive Clinical Genetics Society International Award for 2014 from the British Society of Genetic Medicine. He is president of the African Society of Human Genetics.
Aravinda Chakravarti is Director of the Center for Human Genetics and Genomics, the Muriel G. and George W. Singer Professor of Neuroscience and Physiology, and Professor of Medicine at New York University School of Medicine. He is an elected member of the US National Academy of Sciences, the US National Academy of Medicine and the Indian National Science Academy. He has been a key participant in the Human Genome Project, the International HapMap Project and the 1000 Genomes Project. His research attempts to understand the molecular basis of multifactorial disease. He was awarded the 2013 William Allan Award by the American Society of Human Genetics and the 2018 Chen Award by the Human Genome Organization.
Eileen E. M. Furlong is Head of the Genome Biology Department at the European Molecular Biology Laboratory (EMBL) and a member of the EMBL Directorate. She is an elected member of the European Molecular Biology Organization (EMBO) and the Academia Europaea, and a European Research Council (ERC) advanced investigator. Her group dissects fundamental principles of how the genome is regulated and how it drives cell fate decisions during embryonic development, including how developmental enhancers are organized and function within the 3D nucleus. Her work combines genetics, (single-cell) genomics, imaging and computational approaches to understand these processes. Her research has advanced the development of genomic methods for use in complex multicellular organisms.
Barbara Treutlein is Associate Professor of Quantitative Developmental Biology in the Department of Biosystems Science and Engineering of ETH Zurich in Basel, Switzerland. Her group uses and develops single-cell genomics approaches in combination with stem cell-based 2D and 3D culture systems to study how human organs develop and regenerate and how cell fate is regulated. For her work, Barbara has received multiple awards, including the Friedmund Neumann Prize of the Schering Foundation, the Dr. Susan Lim Award for Outstanding Young Investigator of the International Society of Stem Cell Research and the EMBO Young Investigator Award.
Alexander Meissner is a scientific member of the Max Planck Society and currently Managing Director of the Max Planck Institute (MPI) for Molecular Genetics in Berlin, Germany. He heads the Department of Genome Regulation and is a visiting scientist in the Department of Stem Cell and Regenerative Biology at Harvard University. Before his move to the MPI, he was a tenured professor at Harvard University and a senior associate member of the Broad Institute, where he co-directed the epigenomics programme. In 2018, he was elected as an EMBO member. His laboratory uses genomic tools to study developmental and disease biology with a particular focus on epigenetic regulation.
Howard Y. Chang is the Virginia and D. K. Ludwig Professor of Cancer Genomics at Stanford University and an investigator at the Howard Hughes Medical Institute. He is a physician–scientist who has focused on deciphering the hidden information in the non-coding genome. His laboratory is best known for studies of long non-coding RNAs in gene regulation and development of new epigenomic technologies. He is an elected member of the US National Academy of Sciences, the US National Academy of Medicine, and the American Academy of Arts and Sciences.
Núria López-Bigas is ICREA research Professor at the Institute for Research in Biomedicine and Associate Professor at the University Pompeu Fabra. She obtained an ERC Consolidator Grant in 2015 and was elected as an EMBO member in 2016. Her work has been recognized with the prestigious Banc de Sabadell Award for Research in Biomedicine, the Catalan National Award for Young Research Talent and the Career Development Award from the Human Frontier Science Program. Her research focuses on the identification of cancer driver mutations, genes and pathways across tumour types and in understanding the mutational processes that lead to the accumulation of mutations in cancer cells.
Eran Segal is Professor in the Department of Computer Science and Applied Mathematics at the Weizmann Institute of Science, heading a multidisciplinary laboratory with extensive experience in machine learning, computational biology and analysis of heterogeneous high-throughput genomic data. His research focuses on the microbiome, nutrition and genetics, and their effect on health and disease and aims to develop personalized medicine based on big data from human cohorts. He has published more than 150 publications and received several awards and honours for his work, including the Overton and the Michael Bruno awards. He was recently elected as an EMBO member and as a member of the Israel Young Academy.
Jin-Soo Kim is Director of the Center for Genome Engineering in the Institute for Basic Science in Daejon, South Korea. He has received numerous awards, including the 2017 Asan Award in Medicine, the 2017 Yumin Award in Science and the 2019 Research Excellence Award (Federation of Asian and Oceanian Biochemists and Molecular Biologists). He was featured as one of ten Science Stars of East Asia in Nature ( 558 , 502–510 (2018)) and has been recognized as a highly cited researcher by Clarivate Analytics since 2018. His work focuses on developing tools for genome editing in biomedical research.
Similar content being viewed by others
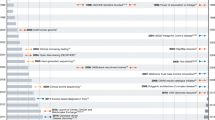
A brief history of human disease genetics
Melina Claussnitzer, Judy H. Cho, … Mark I. McCarthy
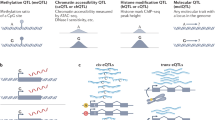
Molecular quantitative trait loci
François Aguet, Kaur Alasoo, … Tuuli Lappalainen
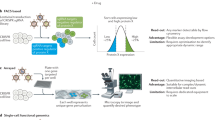
A new era in functional genomics screens
Laralynne Przybyla & Luke A. Gilbert
Making genomics truly equitable
Amy McGuire. For the field of genetics and genomics, the first decade of the twenty-first century was a time of rapid discovery, transformative technological development and plummeting costs. We moved from mapping the human genome, an international endeavour that took more than a decade and cost billions of dollars, to sequencing individual genomes for a mere fraction of the cost in a relatively short time.
During the subsequent decade, the field turned towards making sense of the vast amount of genomic information being generated and situating it in the context of one’s environment, lifestyle and other non-genetic factors. Much of the hype that characterized the previous decade was tempered as we were reminded of the exquisite complexity of human biology. A vision of medicine driven by genetically determined risk predictions was replaced with a vision of precision in which genetics, environment and lifestyle all converge to deliver the right treatment to the right patient at the right time 1 .
As we embark on the third decade of this century, we are now faced with the prospect of being able not only to more accurately predict disease risk and tailor existing treatments on the basis of genetic and non-genetic factors but also to potentially cure or even eliminate some diseases entirely with gene-editing technologies.
These advancements raise many ethical and policy issues, including concerns about privacy and discrimination, the right of access to research findings and direct-to-consumer genetic testing, and informed consent. Significant investment has been made to better understand the risks and benefits of clinical genomic testing, and there has been vigorous debate about the ethics of human gene editing, with many prominent scientists and bioethicists calling for a moratorium on human germline editing until it is proven to be safe and effective and there is broad societal consensus on its appropriate application 2 .
These are all important issues that we need to continue to explore, but as the technologies that have been developed and tested at warp speed over the past two decades begin to be integrated into routine clinical care, it is imperative that we also confront one of the most difficult and fundamental challenges in genomics, in medicine and in society — rectifying structural inequities and addressing factors that privilege some while disadvantaging others. The genomics of the future must be a genomics for all, regardless of ethnicity, geography or ability to pay.
This audacious goal of making genomics truly equitable requires multifaceted solutions. The disproportionate burden of illness and death among racial and ethnic minorities associated with the global COVID-19 pandemic 3 and recent protests against police brutality towards African American citizens 4 have strengthened the antiracism movement and amplified demands for racial equity.
To be part of this movement and effect change will require humility. We must actively listen and learn from each other, especially when it is uncomfortable and our own complicity may be implicated. It will require solidarity and a recognition that we are all connected through our common humanity. And it will require courage. It may seem like a platitude, but it is true that nothing will change unless actual change is made. If we continue to do things as they have always been done, we will end up where we have always been. It is time to step into the discomfort and dare to do something different.
So what can we do differently to make genomics more equitable? I propose three areas where we should focus attention to address this important question. First, we must ensure equitable representation in genomic research. Examining 2,511 studies involving nearly 35 million samples from the GWAS Catalog in 2016, Popejoy and Fullerton found that the vast majority (81%) come from individuals of European descent, with only 5% coming from non-Asian minority populations 5 . This has created an ‘information disparity’ that has an impact on the reliability of clinical genomic interpretation for under-represented minorities 6 . The US National Institutes of Health (NIH) has invested in efforts to increase diversity in genomic research, but to be successful these efforts must be accompanied by serious attention to earning the trust of disadvantaged and historically mistreated populations. This will require, at a minimum, more meaningful engagement, improved transparency, robust systems of accountability, and a commitment to creating opportunities that promote and support a genomics workforce that includes scientists and clinicians from under-represented populations.
It is insufficient to achieve diverse representation in genomic research; however, there must also be equitable access to the fruits of that research. An analysis of the US Centers for Disease Control and Prevention’s 2018 Behavioural Risk Factor Surveillance System found that non-elderly adults from self-identified racial or ethnic minority groups are significantly less likely to see a doctor because of cost than non-elderly white adults 7 . This finding reflects how the structure and financing of health care in the United States perpetuates inequities and contributes to the larger web of social injustice that is at the heart of the problem. Even when socio-economic factors are controlled for, racial disparities in access to genetic services persist 8 . Large-scale, sustained research is needed to better understand and actively address the multitude of factors that contribute to this, including issues related to structural racism, mistrust, implicit and explicit bias, a lack of knowledge of genetic testing, and concerns about misuse of genetic information.
Finally, and perhaps most daunting, we must strive to achieve more equitable outcomes from genomic medicine. Many racial and ethnic minorities disproportionately experience chronic disease and premature death compared with white individuals. Disparities also exist by gender, sexual orientation, age, disability status, socio-economic status and geographical location. Health outcomes are heavily influenced by social, economic and environmental factors. Thus, although providing more equitable access to genomic services and ensuring more equitable representation in genomic research are necessary first steps, they are not enough 9 . Genomics can only be part of the solution if it is integrated with broader social, economic and political efforts aimed at addressing disparities in health outcomes. For genomics to be truly equitable, it must operate within a just health-care system and a just society.
we must strive to achieve more equitable outcomes from genomic medicine
Genome sequencing at population scale
Stacey Gabriel. Twenty years ago, I finished a PhD project that involved laboriously sequencing one gene — a rather complicated one, RET — in a couple of hundred people to catalogue pathogenic variants for Hirschsprung disease. This work required designing primers on the basis of genome sequence data as they were gradually released, amplifying the gene exon by exon (all 20!), running sequencing gels and manually scoring sequence changes. The notion of sequencing the whole genome to catalogue sequence changes was something to wish for in our wildest dreams.
Thanks to great strides in technology and the hard work of geneticists, engineers, epidemiologists and clinicians, much progress has been made; huge numbers of genomes (and exomes) have been sequenced across the world. Disease gene-finding projects such as my graduate work are now done routinely, rather than one gene at a time, using whole-exome or whole-genome sequencing (WGS) in families and affected individuals, enabling the identification of genes and causative mutations in thousands of Mendelian diseases and some complex diseases.
But the real promise of genome sequencing lies in true population-scale sequencing, ultimately at the scale of tens of millions of individuals, whereby genome sequencing of unselected people enables the unbiased, comprehensive study of our genome and the variation therein. It provides a ‘lookup table’ to catalogue disease-causing and benign variants (our ‘allelic series’). The genome sequence should become part of the electronic health record; it is a stable, persistent source of information about a person akin to physical measurements such as weight or blood pressure, exposures such as smoking or alcohol use, and (in many ways better than) self-reported family history.
the real promise of genome sequencing lies in true population-scale sequencing, ultimately at the scale of tens of millions of individuals
What can we learn? What needs to be solved? Even fairly small numbers of genomes aggregated in a consistent and searchable form have enabled a new way to use and interpret genomic data, just in the past couple of years providing a glimpse at the future. Efforts such as gnomAD 10 are a start — this database contains data from more than 15,000 genomes and 125,0000 exomes. With this resource, the frequency of genetic variants within populations is readily available. A clinician interpreting the genome of a patient can ask whether a variant has been observed before. The data provide a starting point for assessing the functional impact of classes of genetic variation and the ability to ask questions about ‘missing’ genetic variation where there is constraint.
Coupled with clinical data, building up population-scale databases of genomic plus clinical information will fuel the application of better risk interpretation using polygenic risk scores (PRSs) 11 . More routine WGS will shorten the ‘diagnostic odyssey’, in which patients suffer through rounds of testing and parents are left uncertain about future reproductive planning. More efficient clinical trials might be built using genomic information. With existing genomic information on all individuals in a health system, trials could be designed in a way that selects individuals most likely to have an event. This enrichment could provide more promising, shorter, smaller and cheaper trial design.
These databases must also rapidly be built in such a way that is representative of the population, representing the actual racial and ethnic diversity, not just what was available as banked sample collections. These are well known to be predominantly European-descent samples and thus preclude application of risk prediction tools in non-white individuals and have limited the ability to find population-specific genetic associations, such as those that have been demonstrated in type 2 diabetes mellitus (T2DM) 12 .
We have to solve important issues — data sharing, privacy and getting the data to scale. Sharing genomic and clinical data is of key importance to drive forward discovery and our understanding of how to use these data in the health-care setting. To do this well and responsibly, trust must be built and maintained through adherence to the rights of privacy, protection and non-discrimination. Progress is being made through the creation of data platforms and the development of frameworks for data protection and sharing; for example, by the work of the Global Alliance for Genomics and Health (GA4GH).
Several large biobanks are already being established to launch population-scale efforts. The UK Biobank is a vanguard programme that contains genotype data, questionnaire-based health and physical measurements on 500,000 individuals and some linkage to their medical records. Other efforts such as the All of Us research programme have been launched with goals directed at true population-based representation, and biobanks that link genomic data to comprehensive medical records in specific health-care systems (for example, Geisinger) or in specific countries or regions (for example, Estonia and Iceland) are also under way.
A big piece of this puzzle is generating comprehensive genome sequence data in these programmes and far beyond. For this aim, large-scale, affordable sequencing is key. No problem, right? Is sequencing not always getting cheaper? The problem is that this assumption is no longer true. We have got to where we are today because for a long time, from 2008 to 2013, sequencing costs dropped exponentially. However, in recent years, the sequencing cost curve has flattened, as is apparent in publicly reported cost estimates provided by the US National Human Genome Research Institute 13 . The cost per megabase of sequence data has remained largely unchanged since around 2016, hovering around a list price of US$0.01 per megabase, which translates to a US$1,000 genome. Gone are the days of our field touting the impressive decrease of cost in comparison with Moore’s law, and this development is worrying.
Some discounting does happen at considerable volume, and whole genomes can be priced in the range of US$500 to US$700. However, large projects (more than 500,000 samples) sequenced at these prices are few and far between, and are generally dependent on pharmaceutical or biotech funding, which can bring with it restrictions on data sharing. It is my belief that a fivefold to sevenfold reduction in total costs is needed to unlock more sequencing at the population scale and, ultimately, for genome sequencing to be more widely applied in the health-care setting. At US$100 per genome, the cost represents less than 1% of the annual average health-care expenditure per person in the United States, and a genome sequence is a one-time investment that can be referenced again and again over the entire lifespan of a person. Getting that cost curve down will be important to inspire health-care systems to adopt genome sequencing routinely.
I see three main drivers that will get us to US$100 per genome: innovation, scale and competition.
Innovation . Generating sequence data requires multiple components, and there are multiple areas ripe for innovation. Sample preparation can be improved through more efficient methods that decrease the labour required, or miniaturization can decrease the cost of the reagents used in library preparation. Developments to decrease data processing costs are also ripe for innovation. Recently, we showed that processing using optimized computing power lowered the time and cost of creating a sequence file by ~50% (S.G., unpublished observations). While decreases in the costs of sample preparation and data processing are important, they represent a small component of the total cost. Roughly 70% of the cost of sequencing a human genome is the sequencing reagent (flow cell) and the instrument. Appreciable cost decrease is made possible only by decreasing these marginal costs, as was demonstrated in the period from 2010 to 2014, when flow-cell densities doubled and sequencing cost dropped by an order of magnitude (US$100 per gigabase to US$10 per gigabase).
Scale . One component of cost is the fixed cost borne by the sequencing centre or the sequencing vendor. With high scale, centres can become more efficient and offset costs such as the costs of personnel, equipment and facilities. Scale can also result in volume discounting of the reagents, although this process is tightly controlled and approached cautiously depending on overall market dynamics.
Competition . Innovation and scale can only achieve so much. The cost of generating the data (the cost per gigabase) dominates and thus must come down considerably. The current market requires alternative options to drive this advance. Presently, the market for short-read sequencing is lacking viable, proven competition that would force flow-cell densities and machine yield to be increased and put pressures on volume discounting. While options for long-read sequencing exist and play a role in particular applications, such as de novo sequencing and structural variant resolution, they are at present far from cost competitive and, therefore, do not apply pressure to bring down the cost of routine WGS.
We need innovation, great economies of scale and/or real competition to come to play in the marketplace. When it comes to sequencing technology, particularly at a large scale, we cannot be complacent and work around the current barriers to realize small gains and one-off wins. This might involve specific types of investment beyond just financial ones; adopting and vetting new technology requires time, creativity, commitment and patience. It is a challenge for our community to take on now. In 5 years’ time, I hope we can look back at the era of the US$100 genome and progress towards real population-scale databases that fuel discovery, enriching our knowledge of the human allelic series and, importantly, the routine use of genomic data in the health-care setting.
A global view of human evolution
Sarah Tishkoff. The past 10 years saw an exponential increase in SNP array and high-coverage WGS data owing to innovations in genomic technologies. It is now possible to generate WGS data from tens of thousands of individuals (for example, GenomeAsia 100K 14 and NIH TOPMed 15 ). An increase in medical biobanks with access to electronic health records (for example, the UK Biobank 16 , the Million Veteran Project 17 and BioBank Japan 18 ) is enabling the mapping of hundreds of genetic associations with complex traits and diseases, as well as phenome-wide association studies 19 to map pleiotropic associations of phenotypes with genes. The genetic associations identified in these and other studies have been used to calculate PRSs for predicting complex phenotypes and risk of diseases.
Yet despite these advances, as of 2019, nearly 80% of individuals in genome-wide association studies (GWAS) were of European ancestries, ~10% were of East Asian ancestries, ~2% were of African ancestries, ~1.5% were of Hispanic ancestries and less than 1% were of other ancestries 20 . There is also a strong European bias in genomic reference databases, such as gnomAD and GTEx . These biases limit our knowledge of genetic risk factors for disease in ethnically diverse populations and could exacerbate health inequities 20 . Furthermore, PRSs that were estimated using European data do not accurately predict phenotypes and disease risk in non-European populations, performing worst in individuals with African ancestry 21 . The lack of transportability of PRSs across ethnic groups is likely due to differences in patterns of linkage disequilibrium and haplotype structure (resulting in different SNPs tagging causal variants), differences in allele frequencies, gene × gene effects and gene × environment effects. It is also possible that the genetic architecture of complex traits and diseases may differ across ethnic groups owing to different demographic histories and adaptation to diverse environments.
Although there have been initiatives to increase inclusion of ethnically diverse populations in human genomics research (for example, the NIH TOPMed 15 and H3Africa consortia), Indigenous populations remain under-represented. Great care must be taken to ensure that genomic research of minority and Indigenous populations is conducted in an ethical manner. This involves establishing partnerships with local research scientists, being sensitive to local customs and cultural concerns, obtaining both community and individual consent, and returning results to communities that participated when possible. In addition, there should be training and capacity building so that genomic research can be conducted locally, where feasible.
A particular area of focus in the future should be developing tools and resources that make genomic data and analyses accessible in low- and middle-income countries. We have to ensure that all people benefit from the genomics revolution and advances in precision medicine and gene editing. Thus, several of the biggest challenges in the next decade will be (1) to increase inclusion of ethnically diverse populations in human genomics research; (2) the generation of more diverse reference genomes using methods that generate long sequencing reads, and haplotype phasing, to account for the large amount of structural variation that likely exists within and between populations; (3) the training of a more diverse community of genomic research scientists; and (4) the development of better methods for accurately predicting phenotypes and genetic risk across ethnically diverse populations and for distinguishing gene × environment effects.
The inclusion of ethnically diverse populations, including Indigenous populations, is also critical for reconstructing human evolutionary history and understanding the genetic basis of adaptation to diverse environments and diets. While there have been a number of success stories for identifying genes of large effect that play a role in local adaptation (for example, lactose tolerance and sickle cell disease (SCD) associated with malaria resistance), identifying signatures of polygenic selection has been considerably more challenging 22 . Genomic signatures of polygenic adaptation are based on the ability to detect subtle shifts in allele frequencies at hundreds or thousands of loci with minor effect on the phenotype of a complex trait and to determine whether that shift is a result of demography or natural selection. A more daunting challenge arises from the same issues of portability of PRSs described earlier — variants associated with a complex trait may not tag well across ethnic groups and/or the genetic architecture of a trait may differ in different populations. Furthermore, it has recently been shown that uncorrected population stratification can result in a false signal of polygenic selection 23 . For example, several studies have identified signatures of polygenic adaptation for height across European populations (selection for increased height in northern Europeans and for decreased height in southern Europeans). However, it was recently shown that these results were influenced by population structure that could not be easily corrected using standard approaches, particularly for SNPs below genome-wide levels of significance 23 . When this analysis was repeated with variants identified in a more homogenous set of individuals of European ancestry from the UK Biobank, these signatures of polygenic adaptation were erased 23 . Thus, methods for detecting polygenic adaptation that are less biased by population structure and by population ascertainment bias will need to be developed in the future. These studies will also benefit from inclusion of more ethnically diverse populations in GWAS and identification of better tag SNPs as described earlier. A challenge of inclusion of minority populations in GWAS is that sample sizes are often small relative to majority populations. However, the high levels of genetic diversity and extremes of phenotypic diversity observed in some populations, particularly those from Africa, make them particularly informative for GWAS. For example, a GWAS of skin pigmentation in fewer than 1,600 Africans was informative for identifying novel genetic variants that affect skin colour, including a previously uncharacterized gene, MFSD12 (ref. 24 ). Thus, genomic studies in the future must make inclusion of minority populations a priority.
A challenge in both GWAS and selection scans has been the identification of causal genetic variants that directly have an impact on variable traits. Most of these variants are in non-coding regions of the genome. The development of high-throughput approaches, such as massively parallel luciferase expression assays to identify gene regulatory regions and high-throughput CRISPR screens in vitro and in vivo to identify functional variants influencing the trait of interest, will be useful 25 . There is also a need to better understand cell type-specific variation and gene regulation at the single-cell level, including response to stimuli such as immune, pharmacological and nutrient challenges, in ethnically diverse populations. However, these approaches are still limited by the need to have informative cell lines. This can be particularly challenging to obtain for Indigenous populations living in remote regions. Improvements in the differentiation of induced pluripotent stem cells (iPS cells) into assorted cell types and into organoids will be important for facilitating functional genomic studies. Establishment of iPS cells and organoids from diverse non-human primate species will also be informative for comparative genomic studies to identify the evolution of human-specific traits such as brain development and cognition. However, iPS cell-derived cells may not accurately reflect the impact of mutations acting on developmental phenotypes, which will require development of more efficient in vivo approaches in model organisms.
Perhaps the biggest revolution in the study of recent human evolutionary history has been the development of methods that make it feasible to sequence and/or obtain targeted genotypes from ancient DNA samples. The generation of high-coverage reference genomes for archaic hominid species such as Neanderthals and Denisovans, located in Eurasia, has made it feasible to identify archaic introgressed segments within the genomes of non-Africans. Some of these regions have been shown to play a role in adaptive traits such as adaptation to high altitude and immune response 26 . Furthermore, there has been an explosion of studies of ancient genetic variation in Europeans within the past 30,000 years that has demonstrated a much more complex model of the peopling of Europe, and the recent evolution of adaptive traits, than previously known from the archaeological record or from studies of modern populations 27 . The biggest challenge has been the inability to get high-quality ancient DNA from regions with a tropical climate, such as Africa and Asia. While there has been success in analysing DNA samples as old as 15,000 years in Africa, which has been informative for tracing recent migration and admixture events 28 , the lack of a more ancient African reference genome makes it very challenging to detect archaic introgression, which currently relies on statistical modelling approaches. Thus, the biggest challenge in the next 10 years will be the successful sequencing of ancient DNA more than 20,000 years old from all regions of the world, so that we may have a better understanding of the complex web of population histories from across the globe.
African genomics — the next frontier
Ambroise Wonkam. To fully meet the potential of global genetic medicine, research into African genomic variation is a scientific imperative, with equitable access being a major challenge to be addressed. Studying African genomic variation represents the next frontier of genetic medicine for three major reasons: ancestry, ecology and equity.
On the basis of a ‘pan-genome’ generated from 910 individuals of African descent, at least 300 million DNA variants (10%) are not found in the current human reference genome 29 , and 2–19% of the genome of ancestral Africans derives from poorly investigated archaic populations that diverged before the split of Neanderthals and modern humans 30 . Neanderthal genome contributions make up ~2% of the genome in present-day Europeans and are enriched for variations in genes involved in dermatological phenotypes, neuropsychiatric disorders and immunological functions 31 . Once technical challenges in sequencing poor-quality DNA have been overcome and approaches to investigate the genomic contribution of African archaic populations have been refined, it is likely that associations between variants in ancient African DNA and human traits or diseases will be found, providing insights that can benefit modern-day humans.
As a consequence of the 300,000–500,000 years of genomic history of modern humans in Africa, ancestral African populations are the most genetically diverse in the world. By contrast, there is an extreme genetic bottleneck, resulting in much less variation, in all non-African populations who evolved from the thousands of humans who migrated out of Africa approximately 70,000 years ago. Current PRSs, which aim to predict the risk for an individual of a specific disease on the basis of the genetic variants that individual harbours, exhibit a bias regarding usability and transferability across populations, as most PRSs do not account for multiple alleles that are either limited or of high frequency among Africans. A GWAS on the genetic susceptibility to T2DM identified a previously unreported African-specific significant locus, while showing transferability of 32 established T2DM loci 32 . In addition, nonsense mutations found commonly among Africans in PCSK9 , which are rare in Europeans 33 , are associated with a 40% reduction in plasma levels of low-density lipoprotein, supporting PCSK9 as a target for dyslipidaemia therapeutics. In the largest GWAS meta-analysis for 34 complex traits, conducted in 14,345 Africans, several loci had limited transferability among cohorts 34 , further illustrating that genomic variation is highest among Africans compared with other populations. As a consequence, linkage disequilibrium is lower in Africans, which improves fine mapping and identification of causative variants. Indeed, while only 2.4% of participants in large GWAS are African individuals, they account for 7% of all associations 35 . Moreover, whole-exome sequencing of nearly 1,000 African study participants of Xhosa ancestry with schizophrenia found very rare damaging mutations in multiple genes 36 , a finding that could be replicated in a Swedish cohort of 5,000 individuals. In comparison, results for the Xhosa cohort yielded larger effect sizes, which shows that for the same number of cases and controls, the greater genetic variation in African populations provides more power to detect genotype–phenotype relationships. Therefore, millions of African genomes must be sequenced, with genotyping and analysis tools optimized for their interrogation.
Greater availability of African genomes will improve our understanding of genomic variation and complex trait associations in all populations but will also support research into common monogenic diseases. The discovery of a single African origin of the SCD mutation, about 5,000–7,000 years ago, not only suggested recent migration and admixture events between Africans and Mediterranean and/or Middle Eastern populations but also enhanced our understanding of genetic variation in general as well as its potential impact on haemoglobinopathies 37 . For example, variants in the HBB -like gene cluster linked with high levels of fetal haemoglobin have been associated with less severe SCD; because the level of fetal haemoglobin is under genetic control, it is amenable to therapeutic manipulation by gene editing 38 . Moreover, knowledge of an individual’s genetic variants can have an impact on secondary prevention of and treatment strategies for SCD. For example, variants in APOL1 and HMOX1 and co-inheritance of α-thalassaemia are associated with kidney dysfunctions 39 ; stroke in SCD is associated with targeted genetic variants used in a Bayesian model; and overall SCD mortality has been associated with circulating transcriptomic profiles. It is estimated that 75% of the 305,800 babies with SCD born each year are born in Africa; SCD in Africa will serve as a model for understanding the impact of genetic variation on common monogenic traits and help to illustrate the multiple layers of genomic medicine implementation.
Greater availability of African genomes will improve our understanding of genomic variation and complex trait associations in all populations
Exploring African genomic diversity will also increase discovery of novel variants and genes for rare monogenic conditions. Indeed, allelic and locus heterogeneity display important differences in African individuals compared with other populations; for example, mutations in GJB2 account for nearly 50% of cases of congenital non-syndromic hearing impairment among Eurasians but are nearly non-existent in Africans, and there is evidence that novel variants in hearing impairment-associated genes are more likely to be found in Africans than in populations of European or Asian ancestries 40 . Higher fertility rate, consanguinity practices and regional genetic bottlenecks will improve novel gene discovery for monogenic diseases in Africa, as well as disease–gene pair curation, and will address existing challenges surrounding database biases and inference of variant deleteriousness, which have led to the misclassification of variants.
Differential population genomic variant frequencies are shaped by natural evolutionary selection as an adaptation to environmental pressures. The African continent follows a North–South axis, which is associated with variable climates and biodiversity, both motors of natural selection. This specific African ecology has shaped genetic variation accordingly, which can have a detrimental or positive impact on health. Obvious examples are variants that cause SCD but confer resistance to malaria 37 , APOL1 variants that are protective against trypanosomes (the parasites that cause sleeping sickness) 41 and variants of OSBPL10 and RXRA that protect against dengue fever 42 . Unfortunately, APOL1 variants also increase susceptibility to chronic kidney disease in populations of African ancestry 39 , 41 . A better understanding of the functional impact of genetic variants specific to African populations, particularly those that have been selected under environmental pressure, and the way they interact with each other is needed and will have a positive impact on genetic medicine practice. Moreover, immunogenetic studies among Africans will further our understanding of natural selection and responses to emerging infectious diseases, such as COVID-19.
The scientific imperative of genomic research of African populations is expected to enhance genetic medicine knowledge and practice in Africa but will face the challenges of overburdened and under-resourced public health-care systems, and often absent ethical, legal and social implication frameworks 43 , requiring international collaboration to be managed. Developing an African genomics workforce will be necessary to meet the major need for research across the lifespan for cohorts of millions of individuals with complex or monogenic diseases. Such endeavours can thrive on the foundation of recently established initiatives such as H3Africa. Indeed, equitable access for Africans is essential if African genomics is to reach its full potential as the next frontier of global genetic medicine.
Decoding multifactorial phenotypes
Aravinda Chakravarti. We live in a time of great technological progress in genomics and computing. And we live in a time when ‘genetics’ is a household word, with a public increasingly adept at understanding its relevance to their own lives. Not surprisingly, the study of genetics is being reinvented, rediscovered and reshaped, and we are beginning to understand the science of human heredity at a resolution that was impossible before.
The most significant genetics puzzle today, in my view, is the dissection of ‘family resemblance’ of complex phenotypes, both for intellectual (raison d'être of genetics) and practical (disease diagnosis and therapy) reasons. We have long known that family resemblance arises from shared alleles, declining as genetic relationship wanes, but the precise molecular components and composition of this resemblance are still poorly understood. At the turn of the twentieth century, the components were a matter of bitter and acrimonious debate 44 between the ‘Mendelians’ and the ‘Biometricians’, until the opposing views were reconciled by Ronald Fisher’s 1918 analysis 45 that complex inheritance could be explained through segregation of many genes, each individually Mendelian. In 1920, its publication delayed by World War I, this notion was elegantly demonstrated by the experimental studies of Altenburg and Muller using truncate wing , an “inconstant and modifiable character” 46 in Drosophila .
Fisher’s model assumed an infinite number of genes additively contributing to a trait, with common genetic variation at each component locus comprising two alleles that differ only slightly in their genetic effects 45 ; these genetic assumptions were quite contrary to what was then known 44 . Throughout the past century, this view matured, as segregation analyses of human phenotypes taught us that — beyond the effects of some major genes — most trait variation was polygenic, modulated by family-specific and random environmental factors 47 . Today, we have empirical evidence from GWAS, which use dense maps of genetic variants on hundreds of thousands of individuals measured for many traits and diseases, that the genetic architecture of most multifactorial traits is from common sequence variants with small allelic differences at thousands of sites across the genome 48 . This replacement of a pan-Mendelian view with a pan-polygenic view of traits is one of the most important contributions of genomics to genetics. Unfortunately, this mapping success has not clarified the number of genes involved, the identity of those genes or how those genes specify the phenotype. Indeed, some have concluded that many of the mapped GWAS loci are unrelated to the core biology of each phenotype 49 . Thus, for a deeper understanding, we need radically different approaches to understand complex trait biology in contrast to merely expanding GWAS in larger and larger samples.
for a deeper understanding, we need radically different approaches to understand complex trait biology
Yet, the most significant biology to emerge from GWAS is that most of the likely trait-causing variants fall outside coding sequences, in regulatory elements, most frequently enhancers 50 , 51 . This important finding has uncovered four new genetic puzzles. First, the non-coding regulatory machinery is vast; how much of this regulation is compromised, and how does it affect phenotypes? Second, regulatory changes affect RNA expression at many genes and protein expression at others; how does a cell ‘read’ these numerous changes as specific signals? Third, how is this coordinated expression response translated into cellular responses affecting phenotypes? Fourth, if specific environmental factors affect the same phenotype, which components do they dysregulate? In my opinion, we need to answer these questions for specific traits and diseases to truly understand their polygenic biology. Finally, these explanations must also answer the question of why some traits are decidedly Mendelian whereas others are not.
The questions of tomorrow will need to focus on four areas: the biology of enhancers and the transcription factors that bind them 51 ; the effect of genetic variation in enhancers 50 ; gene regulatory networks (GRNs) that regulate expression of multiple genes 52 ; and how GRN changes lead to specific cellular responses 53 . Despite many advances, the number of enhancers regulating expression of a specific gene remains unknown. How many enhancers are cell type specific versus ubiquitous? How many are constitutive rather than stage specific? And do they act additively or synergistically in gene expression? Additionally, which cognate transcription factors bind these enhancers, with what dynamics and how are they regulated 54 ? These details of a gene’s ‘enhancer code’ are critical for assessing its relative effect on a trait. Next, how does enhancer sequence variation affect a gene’s activity? Does such variation affect transcription factor binding only or its interaction with the promoter? Is the enhancer variant’s effect evident in all cellular states or only some? Is variation in only one enhancer sufficient to alter gene expression, or are multiple changes in multiple elements necessary?
Additional critical questions include which genes are involved in the core pathway underlying a trait, and how do we identify them 49 ? Elegant work has shown how genes are regulated within integrated modular GRNs, whereby one gene’s product is required in a subsequent step by another gene, with feedback interactions 52 . These GRNs comprise elements from the genome, transcriptome and proteome, with rate-limiting steps that require regulation. As our work on Hirschsprung disease has shown 50 , 53 , a GRN is composed of core genes, is the logic diagram of regulation of a major rate-limiting cellular step, is enriched in coding and enhancer disease variants with disease susceptibility scaling with increasing number of variants, and with disease resulting from effects on its rate-limiting gene product 53 . That is, the GRN integrates the expression of multiple genes. Finally, we need to understand how GRN changes alter cell properties and behaviour. I speculate that rate-limiting steps in GRNs are major regulators of broad cell properties, be they differentiation, migration, proliferation or apoptosis, the cellular integrator of GRN variation. Thus, genetic variation across the genome affects enhancers dysregulating many genes, but only when they dysregulate GRNs through rate-limiting steps do they affect cell and tissue biology 55 . This offers the promise of a mechanistic understanding of human polygenic disease.
The way forward for complex trait biology, including disease, is to shift our approach from reverse to forward genetics, using genome-wide approaches to cell type-specific gene perturbation. I believe we can construct cell-type GRNs en masse, inclusive of their enhancers, transcription factors and feedback or feedforward interactions, to then assay functionally defined variation in phenotypes. But, even this approach will be insufficient. We need to test our success by solving at least a few complex traits completely and demonstrating their veracity using a synthetic biology approach to recapitulate the phenotype in a model system; similarly to the field of chemistry, analysis has to be followed by de novo synthesis. Our genomic technologies are getting up to the task to enable this advance; as geneticists, are we?
Enhancers and embryonic development
Eileen Furlong. The work of my group sits at the interface of genome regulation and animal development, and there have been many exciting advances in both during the past decade. Developmental biology studies fundamental processes such as tissue and organ development and how complexity emerges through the combined action of cell communication, movement and mechanical forces. After the discovery that differentiated cells could be reprogrammed to a naive embryonic stem cell-like state, the past decade has witnessed an explosion in in vitro cellular reprogramming and differentiation studies. Organoids are a very exciting extension of this. The extent to which these fairly simple systems can self-organize and generate complexity 56 is one of the unexpected surprises of the past 5–10 years. The buzz around stem cells has also renewed interest in cellular plasticity in vivo and has uncovered an unexpected degree of transdifferentiation and dedifferentiation 57 . In the mouse heart, for example, cardiomyocytes dedifferentiate and proliferate to regenerate heart tissue when damaged within the first week after birth 58 .
Our understanding of the molecular changes that accompany differentiation has hugely advanced owing to the jump in scale, resolution and sensitivity of next-generation sequencing technologies over the past decade. This has led to a flood of studies in embryonic stem cells, iPS cells and embryos that revealed new concepts underlying genome regulation by measuring transcript diversity, transcription factor occupancy, chromatin accessibility and conformation, and chromatin, DNA and RNA modifications. The future challenge will be to connect this information to the physical characteristics of cells and how they form complex tissues. New technologies that solve many challenges of working with embryos will help, including CRISPR to engineer genomes, optogenetics to perturb proteins, lattice light-sheet and selective plane illumination microscopy to image processes in vivo, and low-input methods to overcome issues with scarce material. Particularly exciting to me are recent advances in single-cell genomics, which, although they are in their early days, will dramatically change the way we study embryogenesis. Many new insights have already emerged, including the discovery of unknown cell types and new developmental trajectories for well-established cell types. Even the concept of ‘cell identity’ has come into question.
Cell identities are largely driven by transcription factors, which act through cis -regulatory elements called ‘enhancers.’ One of the most exciting unsolved mysteries, in my opinion, is how enhancers relay information to their target genes. The textbook view of enhancers is of elements with exclusive function that regulate a specific target gene through direct promoter interactions, which occur sequentially if multiple enhancers are involved. However, emerging concepts in the past decade question many of these ‘dogmas’. Some enhancers have dual functions, whereas others may even regulate two genes. Enhancer–promoter communication is now viewed in the light of spatial genome organization, including topologically associating domains (TADs) and membraneless nuclear microcompartments (that is, hubs or condensates) 59 . Being present within the same TAD likely increases the frequency of enhancer–promoter interactions, but how a specific enhancer finds its correct promoter within a TAD, or when TADs are rearranged 60 , 61 , remains a mystery. Hubs or condensates are dynamic microcompartments 62 that contain high local concentrations of proteins, including transcription factors and the transcriptional machinery. One potential implication of condensates is that enhancers may not need to ‘directly’ touch a gene’s promoter to regulate transcription — rather, it may be sufficient to come in close proximity within the same condensate. Presumably, once proteins reach a critical concentration, transcription will be initiated. While this model fits a lot of emerging data, there are still many open questions. What is the required distance between an enhancer and a promoter to trigger transcription? Does this distance differ for different enhancers 63 depending on their transcription factor–DNA affinities? Do different chromatin environments 64 influence the process? At some loci, mutation of a single transcription factor-binding site in a single enhancer can have dramatic effects on gene expression and development. It is difficult to reconcile such cases with a shared condensate model, as other proteins bound to the enhancers and promoter should still phase separate. By contrast, there are many examples where mutation of a single transcription factor-binding site, or even an entire enhancer, has minimal impact on the expression of a gene. These observations suggest that there may be different types of loci, with requirements for different types of chromatin topologies and local nuclear environments, which will be important to tease apart in the coming years.
The genetic dissection of model loci in the 1990s and the first decade of the twenty-first century led to much of our understanding of how genes are regulated. The power of genomics in the past few decades has captured regulatory information for all genes genome-wide, providing more unbiased views of regulatory signatures, leading to new models of gene regulation. What is missing is empirical testing at a large scale. A major challenge is to move to more systematic in vivo functional dissection in organisms. CRISPR-based pooled screens have advanced the interrogation of genomic regions in cell culture systems. However, scaling functional assays in embryos remains a huge challenge. The task is enormous — even long-standing model organisms, such as Drosophila and mice, lack knockout strains for all protein-coding genes, and the number of regulatory elements is at least an order of magnitude higher. There has been little progress in developing scalable methods to quantify the contribution of a transcription factor’s input to an enhancer’s activity, and gene expression, in embryos. More systematic unbiased data will uncover more generalizable regulatory principles, increase our predictive abilities of gene regulation and developmental programmes, and enhance our understanding of the impact of genetic variation.
A major challenge is to move to more systematic in vivo functional dissection in organisms
Perhaps the most promising and exciting prospects in the coming years are to use single-cell genomics, imaging and the integration of the two to dissect the amazing complexity of embryonic development. Single-cell genomics can reveal information about developmental transitions in a way that was unfeasible before. When combined with temporal information, such data can reconstruct developmental trajectories 65 , 66 and identify the regulatory regions and transcription factors likely responsible for each transition 67 . The scale and unbiased nature of the data, profiling tens to hundreds of thousands of cells, provides much richer information than anyone envisaged just 5 years ago, bringing a new level of inference and causal modelling. The ability to measure single-cell parameters in situ (called ‘spatial omics’) will be transformative in the context of developing embryos to reveal the functional impact of spatial gradients, inductive signals and cell–cell interactions, and to move to digital 4D embryos. Combining these approaches with genetic perturbations holds promise to decode developmental programmes as they unfold. Will this bring us to a predictive understanding of the regulatory networks driving embryonic development during the next decade? ‘Simple’ model organisms are a fantastic test case to determine the types and scale of data required and to develop the computational framework to build predictive networks. The systematic functional dissection of gene regulation and true integration of single-cell genomics with single-cell imaging will bring many exciting advances in our understanding of the programmes driving embryonic development in the coming years.
Spatial multi-omics in single cells
Barbara Treutlein. Incredibly, the first single-cell transcriptome was sequenced just over a decade ago 68 ! Since this milestone, transcriptomes of millions of cells have been sequenced and analysed from diverse organisms, tissues and other cellular biosystems, and these maps of cell states are revolutionizing the life sciences. The technologies and associated computational methods have matured and been democratized to such an extent that nearly all laboratories can apply the approach to their particular system or question.
Of course, the transcriptome is not enough, and protocols have already been developed to measure chromatin accessibility, histone modifications, protein abundances, cell lineages and other features linked to genome activity in single cells 69 . Currently, many studies use dissociation-based single-cell genomics methods, where the spatial context is disrupted to facilitate the capture of single cells for downstream processing. Methods are improving to measure genomic features in situ 70 , as well as to computationally map features to spatial contexts 71 , 72 . The stage is set for the next phase of single-cell genomics, where spatial registration of multimodal genome activity across molecular, cellular and tissue or ecosystem scales will enable virtual reconstructions with extraordinary resolution and predictive capacity. These virtual maps will rely on multi-omic profiling of healthy and perturbed tissues and organisms, which presents major challenges and opportunities for innovation.
Cell throughput remains a challenge, and it is unclear what role dissociation-based single-cell sequencing protocols will play in the future. These protocols are fairly easy to implement, and laboratories around the world can execute projects with tens of thousands of cells analysed per experiment. However, there are scenarios in which measuring millions of cells per experiment would be desired, such as in perturbation screens. Combinatorial barcoding methods push cell-throughput boundaries 73 ; however, it is unclear how to scale full transcriptome sequencing economically to millions of cells using current sequencing technologies. ‘Compressed sensing’ modalities — whereby a limited, selected and/or random number of features are measured per cell, and high-dimensional feature levels are recovered through inference or similarity to a known reference — provide an interesting possibility to increasing cell throughput 74 .
Most single-cell transcriptome protocols are currently limited to priming the polyadenylation track present on all cellular mRNAs; however, this approach leads to biased sampling of highly expressed mRNAs. Clever innovations for random or targeted RNA enrichment could be a way to build up composite representations of cell states. Image-based in situ sequencing methods provide a means for increasing the number ofcells measured per experiment, as millions of cells can be imaged without a substantial increase in financial cost, although imaging time is a limiting factor. There remains a lot of room for experimental and computational optimizations to measure the transcriptome, random barcodes, DNA conformations and protein abundances from the micrometre scale to the centimetre scale spatially, and it will be interesting to see how methods for spatial registration advance over the next 5 years.
Currently, most high-throughput measurements are performed on cell suspensions or on intact tissues using one modality. That said, studies are emerging that measure several features from the same cell; for example, mRNA and chromatin accessibility 75 or mRNA and lineage 76 . To build virtual maps, independent measurements from different cells can be integrated with use of data integration tools 77 , although it can be difficult to align cell states across modalities in particular in developing systems. Therefore, the ultimate goal is to directly measure as many features as possible (for example, RNA, lineage, chromatin, proteins and DNA methylation) in the same cell 78 , ideally with spatial resolution. Furthermore, combining genetic and pharmacological perturbation screens with single-cell multi-omic measures will be informative to understand cell state landscapes and underlying regulatory networks for each cell type. The CRISPR–Cas field continues to develop creative tools for precise single-locus editing and other manipulations 79 , and incorporation of these toolkits with single-cell sequencing readouts will certainly bring new mechanistic insight.
Life forms are inherently dynamic, and each cell has a story to tell. Static measurements do not provide sufficient insight into the mechanisms that give rise to each cell state observed in a tissue. Computational approaches to stitch together independent measurements across time can be used to reconstruct potential histories; however, these are indirect inferences. Long-term live imaging in 2D cultures using confocal microscopy and in 3D tissues using light-sheet microscopy provides morphology, behaviour, location and, in some cases, molecular information on the history of a cell. Indeed, such long-term imaging experiments revealed that cell fates or states can be predicted from cell behaviour across many generations 80 . Cell tracking combined with end point single-cell genomics experiments can help to understand how cell states came to be; however, these experiments lack molecular resolution of the intermediates. There are strategies using CRISPR–Cas systems to capture highly prevalent RNAs inside cells at given times and insert these RNAs into DNA for storage and subsequent readout 81 . Together with live tracking and end-point single-cell genomics, such methods could provide unprecedented insight into cell histories.
My vision is that the emerging technologies described above can be applied to human 2D cell culture and 3D organoid biosystems to understand human development and disease mechanisms. My team and others are working to build virtual human organs that are based on high-throughput, multimodal single-cell genomics data. Organoid counterparts provide opportunities to perturb the system and understand lineage histories. Together, the next generation of single-cell genomics methods and human organoid technologies will provide unprecedented opportunities to develop new therapies for human disease.
the next generation of single-cell genomics methods and human organoid technologies will provide unprecedented opportunities
Unravelling the layers of the epigenome
Alexander Meissner. Around 1975, the idea that 5-methylcytosine could provide a mechanism to control gene expression gained traction, despite little knowledge of its genomic distribution or the associated enzymes 82 . With similarly limited genomic information or knowledge of the players involved, the histone code hypothesis was put forward in 2000 to explain how multiple different covalent modifications of chromatin may be coordinated to direct specific regulatory functions 83 . Tremendous progress has been made since, and the list of core epigenetic regulators that have been discovered and characterized seems largely complete 84 .
DNA sequencing has continued to dominate the past decade and contributed to an exponential growth of genome-wide maps of all layers of regulation. In the early days, individual CpG sites could be measured by restriction enzymes, whereas now we have generated probably well over a trillion cytosine methylation measurements. An equally astonishing number of genome-wide data sets have been collected for transcriptomes, histone modifications, transcription factor occupancy and DNA accessibility. Furthermore, the number of single-cell transcriptome and epigenome data sets continues to grow at an unprecedented pace.
On the basis of this overabundance of data across many normal and diseased cell states, for instance, we now clearly understand the non-random distribution of cytosine methylation across many different organisms. These maps have helped to refine our understanding of its relationship to gene expression, including the realization that only a few promoters are normally controlled via this modification, whereas gene bodies are actively targeted, and most dynamic changes occur at distal regulatory sites. Similar insights exist for many core histone modifications, and, in general, we have an improved appreciation of the epigenetic writers, readers and erasers involved. Over the past decade, we have seen substantially integrated and multilayered epigenomic analyses that provide a fairly comprehensive picture of epigenomic landscapes, including their dynamics across development and disease.
Additional innovation is now needed around data access and sharing. As noted, there is certainly no shortage of data, but to enable individual researchers to generate and verify hypotheses quickly improved tools are required to access and browse these data. Over the past decade, large coordinated projects such as ENCODE , the Roadmap Epigenomics Project and Blueprint Epigenome have initiated such efforts, but it remains a reality that data are not at everyone’s fingertips quite yet.
Moreover, despite decades of steady and recently accelerated progress, many important questions remain regarding the molecular coordination and developmental functions of these epigenetic modifications. For instance, cytosine methylation at gene bodies has been preserved for more than a billion years of evolution and yet its precise function is still under investigation. How and why did genomic methylation switch to a global mechanism in vertebrates compared with the selected methylation observed in invertebrates? What is the precise function of this modification in each of its regulatory contexts, and how are its ubiquitously acting enzymes recruited to specific sites in the genome? The latter is particularly timely given recent observations that enhancers, but also some repetitive elements, show ongoing recruitment of both de novo methylation and demethylation activity. Moreover, extraembryonic tissues show redirected activity that shares notable similarities with the long observed altered DNA methylation landscape found across most cancer types 85 . Lastly, it is abundantly clear that DNA methylation is essential for mammalian development; but despite us knowing this for nearly three decades, it is not clear how and why developing knockout embryos die. The specific developmental requirements are also largely true for many histone-modifying enzymes; however, it remains incompletely understood how exactly these modifications interact to support gene regulation.
A decade ago it seemed likely that we would answer questions such as these using newly gained sequencing power as a potent tool for generating hypotheses. However, for the most part, epigenomic analyses have expanded a highly valuable, but still largely descriptive, understanding of numerous epigenetic layers. So one may ask, what is different now and why should we expect to answer these questions in the coming years?
Technological innovation has always played a key role in biology, and some broadly applicable, recent breakthroughs will enable us to drive progress in the coming years. These include the transfer of the bacterial innate immunity CRISPR–Cas system as a universal genome-targeting tool 86 as well as for base editing, epigenome editing and various genome manipulations. Similarly, new fast-acting endogenous protein degradation systems have been developed that further enhance our ability to probe for precise function 87 . The past decade also saw major improvements in imaging technologies as well as cell and molecular biology, moving from the 2D space into the 3D space with both organoid cell culture models 88 and chromosome conformation capture approaches for exploring nuclear organization 89 .
Another major shift included the reappreciation that membraneless organelles are a widespread mechanism of cellular organization 90 . In particular, there have been many advances in our understanding of how condensates form and function, including for transcriptional regulation. Together with known properties of modified histones on DNA and the fact that many epigenetic regulators also contain intrinsically disordered regions, it is reasonable to assume that these physical properties will have a major impact on our understanding of chromatin. Importantly, changes in topology have been linked to disease 91 , and similar connections have been reported recently for condensates 92 . This will likely be an exciting area to follow in the coming years.
there have been many advances in our understanding of how condensates form and function, including for transcriptional regulation
Lastly, our research continues to be more and more reliant on multidisciplinary skills, with mathematics, physics, chemistry and computer science playing an ever-more central role in biology, which will require some rethinking in training and institutional organization to accomplish our goals. Going forward, we will need more functional integration, which in part due to the aforementioned selected discoveries is now very tractable. In particular, more refined perturbation of gene activity, which for many chromatin regulators should be separated into catalytic and regulatory functions, together with readouts at multiple levels of resolution will bring us closer to the insights needed. We recently exemplified this with a pipeline that explores epigenetic regulator mutant phenotypes at single-cell resolution 93 . From these studies, we may be able to understand how epigenetic regulators interact with the environment to influence or protect the organismal phenotype, connecting detailed molecular genetics to classical theories of epigenetic phenomena.
As we approach the 100-year anniversary of the detection of 5-methylcytosine in DNA 94 , it seems we can hope to declare at least for some layers of the epigenome that we fully understand the rules under which they operate. This may enable the exploration of more precise therapeutic interventions, for instance by redirecting chromatin modifiers rather than blocking their universal catalytic activities, which are shared between normal and diseased states. Of course, looking back at predictions made just 10 years ago 95 , one should expect many additional unforeseen advances that are just as difficult to predict now as they were back then.
Long non-coding RNAs: a time to build
Howard Chang. Long non-coding RNAs (lncRNAs) are the dominant transcriptional output of many eukaryotic genomes. Although studies over the past decade have revealed diverse mechanisms and disease implications for many lncRNAs, the vast majority of lncRNAs remain mysterious. The fundamental challenge is that we lack the knowledge to systematically transform lncRNA sequence into function. Progress in the next decade may come from a paradigm shift from ‘reading’ to ‘writing’ lncRNAs.
Gene regulation was once thought to be the exclusive province of proteins. Intense efforts for disease diagnosis and treatment focused almost entirely on protein-coding genes and their products, ignoring the vast majority of the genome. Even at the time of the completion of the Human Genome Project, only a handful of functional lncRNAs were known that silenced the expression of neighbouring genes. Thus, it was widely believed that the genome contained mostly ‘junk’, which sometimes made RNA as transcriptional noise.
The human genome is currently estimated to encode nearly 60,000 lncRNAs, ranging from several hundred to tens of thousands of bases, that apparently do not function by encoding proteins 96 . Studies over the past decade discovered that many lncRNAs act at the interface between chromatin modification machinery and the genome. Specific lncRNAs can act as guides, scaffolds or decoys to control the recruitment of specific chromatin modification enzymes or transcription factors to DNA or their dismissal from DNA 97 . lncRNAs can activate as well as silence genes, and these RNAs can target neighbouring genes as a function of local chromosomal folding (in cis ) or at a distance throughout the genome (in trans ). Detailed dissections of individual lncRNAs have revealed that lncRNAs are composed of modular RNA motifs that enable one lncRNA to connect proteins that read, write or erase specific chromatin marks. These findings have galvanized substantial excitement about lncRNAs; laboratories around the world are now investigating the roles of lncRNAs in diverse systems, ranging from control of flowering time in plants to mutations in human genetic disorders.
Nonetheless, the notable progress to date can be viewed as anecdotal — each lncRNA is its own story. When a new lncRNA sequence is recognized in a genome database or RNA profiling experiment, we are still in the dark about what may happen to the cell or organism (if anything) when the lncRNA is removed. Indeed, efforts to ‘read’ lncRNAs have been the dominant experimental strategy over the past two decades. Systematic efforts in the ENCODE, FANTOM and emerging cell atlas consortia have mapped the transcriptional landscape, transcript isoforms and, more recently, single-cell expression profiles of lncRNAs. These powerful data are now combined with genome-scale CRISPR-based methods to inactivate tens of thousands of lncRNAs, one at a time, to observe possible cell defects 98 , 99 . However, many challenges remain. Positive hits require further exploratory studies to define possible mechanisms of action, and we lack a principled strategy to combine lncRNA knockouts to address genetic redundancy and compensation.
A potentially fruitful and complementary direction is the pivot from ‘reading’ to ‘writing’ long RNA scripts. On the basis of the systematic dissection of RNA sequences and secondary structures in lncRNAs, we and others believe that the information in lncRNAs resembles that on a billboard (in which keywords and catchphrases are repeated) rather than a finely honed legal document (where every comma counts). Small units of RNA shapes are repeated within lncRNAs to build up the meaning in the lncRNA billboard, but these RNA shapes can be rearranged in different orders or locations without affecting meaning. These insights have allowed scientists to recognize lncRNA genes from different species that perform the same function even though the primary sequences bear little similarity 100 . Moreover, investigators were able to strip down lncRNAs to their essential ‘words’, composed of these key repeating shapes and one-tenth the size of the original lncRNA, which still functioned in vivo to control chromatin state over a whole chromosome 100 , 101 . Finally, it is now possible to successfully create synthetic lncRNAs. By adding RNA shapes to carefully chosen RNA templates, investigators are starting to create designer lncRNAs that can regulate chromatin in vivo 100 , suffice to partly rescue the physiological lncRNA gene knockout 102 , or target RNAs to specific cytotopic locations within the cell 103 , 104 .
The shift from reading to writing lncRNAs will challenge us on the technical front, leading to potential transformative technologies. Current technologies for massively parallel reporter gene assays are built on short sequence inserts. A plan to build tens of thousands of synthetic lncRNAs will require accurate long DNA or RNA synthesis. These designer sequences will need to be placed into the appropriate locations in the genome and controlled to have proper developmental expression, splicing pattern and RNA chemical modifications. Landmark studies using the XIST lncRNA, which normally silences the second X chromosome in female cells, to silence the ectopic chromosome 21 in Down syndrome cells highlight the biomedical promise of such an approach 105 .
As the field develops technologies for large-scale creation and testing of synthetic lncRNAs, we can rigorously test our understanding of the information content in the language of RNA sequences and shapes. The next decade promises to be an exciting time for building non-coding RNAs and to create entirely new tools to manipulate gene function for biology and medicine.
FAIR genomics to track tumorigenesis
Núria López-Bigas. Cancer research is one of the fields that has probably benefited the most from the technological and methodological advances of genomics. In the span of less than two decades, the field has witnessed an incredible boost in the generation of cancer genomic, epigenomic and transcriptomic data of patients’ tumours, both in bulk and more recently at the single-cell level. My dream as a cancer researcher is to have a full understanding of the path that cells follow towards tumorigenesis. Which events in the life of an individual, a tissue and a particular cell lead to the malignant transformation of some cells? Of course I do not expect to have a deterministic answer, as this is not a deterministic process. Instead we should aim for a quantitative or probabilistic understanding of the key events that drive tumorigenesis. We have solid epidemiological evidence showing that smoking increases the probability of lung cancer, exposure to the Sun raises the probability of developing melanoma and some anticancer treatments increase the probability of secondary neoplasms. But which specific mechanisms at the molecular and cellular levels influence these increases?
One first clear goal of cancer genomics is to catalogue all genes involved in tumorigenesis across different tissues. Although this is a daunting task, it is actually feasible 106 . By analysing the mutational patterns of genes across tumours, one can identify those with significant deviations from what is expected under neutrality, which indicates that these mutations provide a selective advantage in tumorigenesis and are thus driver mutations. We can imagine a future in which through the systematic analysis of millions of sequenced tumour genomes this catalogue or compendium moves closer and closer to completion. For this to happen, not only do we need genome sequencing to expand — this process is already in motion in research, clinical settings and the pharmaceutical industry — but more importantly the resulting data must be made FAIR (findable, accessible, interoperable and reusable) 107 . To this end, consortia and initiatives that promote, catalyse and facilitate the sharing of genomic data, such as the Beyond 1 Million Genomes consortium, the GA4GH or the cBioPortal for Cancer Genomics , are necessary.
Of note, cataloguing genes and mutations involved in cancer development, albeit a very important first step, is still far from the final goal of understanding how and under which conditions they drive tumorigenesis. Framing cancer development as a Darwinian evolutionary process helps me to navigate the path towards this final objective. As is true of any Darwinian process, its two key features are variation and selection. Thanks to the past 15 years of cancer genomics, we now have a much better grasp of the origin of somatic genetic variation between cells across different tissues. The study of the variability in the number, type and genomic distribution of mutations across tumours provides a window into the life history of cells across the somatic tissues of an individual 108 , 109 . In addition, recent studies sequencing the genome of healthy cells in different tissues 110 , 111 , 112 have shown that mutations accumulate in hundreds and thousands in our cells in normal conditions over time. These studies have also detected positive selection in some genes across healthy tissues. Hence, positive selection is a pervasive process that operates not only in tumorigenesis but also in healthy tissues, where it is a hallmark of somatic development of skin, oesophagus, blood and other tissues. Take, for example, clonal haematopoiesis: it results from a continuous Darwinian evolutionary process in which over time (with age) some haematopoietic cells harbouring mutations in certain blood development genes, such as DNMT3A and TET2 , outcompete other cells in the compartment 113 , 114 . This process is part of normal haematopoietic development. Problems arise only when this process gets out of control, leading to leukaemia in the case of blood, or a malignant tumour in solid tissues. Why is it only in rare cases that this ubiquitous interplay between variation and selection becomes uncontrollable and results in full-blown tumorigenesis? Which events, beside known tumorigenic mutations, drive this process?
we now have a much better grasp of the origin of somatic genetic variation between cells across different tissues
If we have learnt something in recent years, it is that virtually all tumours harbour driver mutations 115 , 116 , 117 , implying that driver genomic events are necessary. However, they are clearly not sufficient for tumorigenesis to occur. So, what are these other triggers of the tumorigenic process? What happens in the lung cells of a smoker or in the haematopoietic cells of a patient treated with chemotherapy that increases their chances to become malignant? Epigenetic modifications and changes in selective constraints, such as evolutionary bottlenecks, for example, at the time of chemotherapy, may be part of the answer.
For the near future, my dream is to see a further increase in FAIR cancer genomics data to help us disentangle the step-by-step game of variation and selection in our tissues that leads to tumorigenesis and likely other ageing-related diseases.
Integrating genomics into medicine
Eran Segal. The past 20 years in genomics have been extraordinary. We developed high-throughput sequencing and learned how to use it to efficiently sequence full genomes and measure gene expression and epigenetic marks at the genome-wide scale and even at the single-cell level 118 . Using these capabilities, we created unprecedented catalogues of novel genomes, functional DNA elements and non-coding RNAs from all kingdoms of life 119 . But — perhaps with the exception of cancer 120 and gene therapy for some monogenic diseases 121 — genomics has yet to deliver on its promise to have an impact on our everyday life. For example, drugs and diagnostics are still being developed in the traditional way, with screening assays to find lead compounds for targets typically arising from animal studies, without involving genomics in any of the steps. Moreover, when the global COVID-19 pandemic hit, the genome of the spreading severe acute respiratory syndrome coronavirus 2 (SARS-CoV-2) was rapidly sequenced, but why some infected individuals exhibit severe disease and others do not remains unknown.
Indeed, our next challenge is to translate the incredible resources and technologies developed in genomics into an improved understanding of health and disease. This improved understanding should transform the field of medicine to use genomics in its transition to personalized medicine, which promises individualized treatment by targeting the right medication to the right person at the right time on the basis of that person’s unique profile. By continuing to focus on more and more measurements and the creation of more atlases and catalogues, we run the danger of drowning in ever-growing amounts of data and correlative findings. Walking down this path can lead to an endless endeavour, as bulk measurements can always be replaced with single-cell ones, or measures at higher temporal and spatial resolution, across more conditions and wider biological contexts.
Instead, we should use genomics to tackle big unanswered questions such as what causes the variation that we see across people in phenotypes, disease susceptibility and drug responses? What is the relative contribution of genetic, epigenetic, microbiome and environmental factors? How are their effects mediated, and what would be the effect of different interventions? Ultimately, we should strive to use genomics to generate actionable and personalized insights that lead to better health. We are now at an inflexion point in genomics that allows us for the first time to apply it to study human biology and realize these ambitious aims 122 .
At the cellular level, we can use iPS cells from patients to derive cellular models of multiple diseases and prioritize treatments based on measuring both their cellular and molecular response (for example, gene expression and epigenetics) to existing drugs and drug combinations. We can even use massively parallel assays to separately measure the effect of each of tens of thousands of rationally designed mutations, including patient-specific mutations, as we have done, for example, in testing the effect of all clinically identified mutations in TP53 on cellular function 123 . Measuring the molecular effects of directed mutations in genes encoding transcription factors and signalling molecules and in other genes can reveal the underlying pathways and regulatory networks of the disease studied and identify putative therapeutic targets. The application of such approaches to fields that are still poorly understood, such as neurodegenerative diseases, can be particularly impactful.
But we can be much more ambitious and directly profile large cohorts of human individuals using diverse ‘omics’ assays. As molecular changes typically precede clinical disease manifestations, longitudinal measurements coupled with clinical phenotyping have the potential of identifying novel disease diagnostics and therapeutic targets. Indeed, biobanks that track large samples of hundreds of thousands of individuals have recently emerged and are proving highly informative 124 . However, at the molecular level their focus has thus far been on genetics. Technological advances and cost reductions now allow us to obtain much deeper person-specific multi-omic profiles that include transcriptome, proteome, methylome, microbiome, immune system and metabolome measurements. Having these data on the same individual and at multiple time points can reveal which omic layer is more perturbed and informative for each disease and identify associations between molecular markers and disease.
The challenge in using such observational data from human cohorts is to identify which of the associations are causal. One way to address this is to wisely select the nature and type of the associations studied. For example, in working with microbiome data, we can move from analyses at the level of species composition to analyses at the level of SNPs in bacterial genes. Such associations are more specific and more likely to be causal, as in the case of a SNP in the dadH bacterial gene, which correlated with metabolism of the primary medication to treat Parkinson disease and the gut microbiota from patients 125 . Another approach is to use longitudinal measurements and separation of time to emulate target trials from observational data 126 . For example, we can select distinct subsets from the cohort that match on several known risk factors (for example, age or body mass index) but differ on a marker of interest (for example, expression of a gene or presence of an epigenetic mark), and compare future disease onset or progression in these two populations. Similarly, retrospective analysis of baseline multi-omic measurements from participants in randomized clinical trials may identify markers that distinguish responders from non-responders and be used for patient stratification or for identifying additional putative targets.
Ultimately, biomarkers identified from observational cohorts need to be tested in randomized clinical trials to establish causality and assess efficacy. In the case of microbial strains extracted from humans, we may be able to skip animal testing and go directly to human trials. In other cases, such as when human genes are being manipulated, we will need to start with cell culture assays and animal testing before performing clinical trials in humans. However, in all cases, tested omic targets should have already shown associations in human individuals, thus making them more likely to be relevant and succeed in trials, as is the case with drug targets for which genetic evidence links them to the disease 127 .
Beyond these scientific challenges, there is the challenge of engaging the public and diverse ethnic and socio-economic groups to participate in such large-scale multi-omic profiling endeavours even before we can present them with immediate benefits. We can start with incentives in the form of informational summary reports of the data measured and gradually move towards carefully and responsibly conveyed actionable insights as we learn more.
Overcoming the aforementioned challenges is not an easy task, but with the breathtaking advances that genomics has undergone in the past two decades, the time may be right to tackle them. Success can transform genomics from being applied mostly in research settings to having it become an integral and inseparable part of medicine.
CRISPR genome editing enters the clinic
Jin-Soo Kim. In the past several years, genome editing has come of age 128 , in particular because of the repurposing of CRISPR systems. Genomic DNA can be modified in a targeted manner in vivo or in vitro with high efficiency and precision, potentially enabling therapeutic genome editing for the treatment of both genetic and non-genetic diseases. All three types of programmable nucleases developed for genome editing, namely zinc-finger nucleases, transcription activator-like effector nucleases and CRISPR nucleases, are now under clinical investigation. In the next several years, we will be able to learn whether these genome-editing tools will be effective and safe enough to treat patients with an array of diseases, including HIV infection, leukaemia, blood disorders and hereditary blindness, heralding a new era in medicine.
If the history of the development of novel drugs or treatments such as gene therapy and monoclonal antibodies is any guide, the road to therapeutic genome editing is likely to be bumpy but ultimately worth travelling. Key questions related to medical applications of programmable nucleases concern their mode of delivery, specificity, on-target activity and immunogenicity. First, in vivo delivery (or direct delivery into patients) of genes or mRNAs encoding programmable nucleases or preassembled Cas9 ribonucleoproteins can be a challenge, given the large size of these nucleases. Ex vivo (or indirect) delivery is, in general, more efficient than in vivo delivery but is limited to cells from blood or bone marrow, which can be collected with ease, edited in vitro and transfused back into patients. Ongoing developments of nanoparticles and viral vectors are expected to enhance and expand in vivo genome editing in tissues or organs not readily accessible with current delivery systems, such as the brain.
Second, programmable nucleases, including CRISPR nucleases, can cause unwanted on-target and off-target mutations, which may contribute to oncogenesis. Several cell-based and cell-free methods have been developed to identify genome-wide CRISPR off-target sites in an unbiased manner 129 , 130 , 131 . But it remains a challenge to validate off-target activity at sites with low mutation frequencies (less than 0.1%) in a population of cells, owing to the intrinsic error rates of current sequencing technologies. Even at on-target sites, CRISPR–Cas9 can induce unexpected outcomes such as large deletions of chromosomal segments 132 . It will be important to understand the mechanisms behind the unusual on-target activity and to measure and reduce the frequencies of such events.
Last but not least, Cas9 and other programmable nucleases can be immunogenic, potentially causing undesired innate and adaptive immune responses. In this regard, it makes sense that initial clinical trials have focused on ex vivo delivery of Cas9 ribonucleoproteins into T cells or in vivo gene editing in the eye, an immunologically privileged organ. Cas9 epitope engineering or novel Cas9 orthologues derived from non-pathogenic bacteria may avoid some of the immune responses, offering therapeutic modalities for in vivo genome editing in tissues or organs with little or no immune privilege.
Base editing 133 , 134 and prime editing 135 are promising new approaches that may overcome some of the limitations of nuclease-mediated genome editing. Base editors and prime editors are composed of a Cas9 nickase, rather than the wild-type Cas9 nuclease, and a nucleobase deaminase and a reverse transcriptase, respectively. Because a nickase, unlike a nuclease, produces DNA single-strand breaks or nicks, but not double-strand breaks (DSBs), base editors and prime editors are unlikely to induce large deletions at on-target sites and chromosomal rearrangements resulting from non-homologous end joining (NHEJ) repair of concurrent on-target and off-target DSBs. Furthermore, when it comes to gene correction rather than gene disruption, these new types of gene editors are much more efficient and ‘cleaner’ than DSB-producing nucleases because they neither require donor template DNA nor rely on error-prone NHEJ; in human cells, DSBs are preferentially repaired by NHEJ, leading to small insertions or deletions (indels), rather than by homologous recombination involving donor DNA.
Base editors and prime editors are also well suited for germline editing and in utero editing (that is, gene editing in the fetus), which should be done with caution, in full consideration of ethical, legal and societal issues. In principle, CRISPR–Cas9 can be used for the correction of pathogenic mutations in human embryos; however, donor DNA is seldom used as a repair template in human embryos 136 . Recurrent or non-recurrent de novo mutations are responsible for the vast majority of genetic diseases. Cell-free fetal DNA in the maternal blood can be used to detect these de novo mutations in fetuses, which are absent in the parents. Some de novo mutations are manifested even before birth, leading to miscarriage, disability or early death after birth; it is often too late and inefficient to attempt gene editing in newborns. These mutations could be corrected in utero using base editors or prime editors without inducing unwanted indels and without relying on inefficient homologous recombination. Compared with germline editing or preimplantation genetic diagnosis, in utero editing, if proven safe and effective in the future, should be ethically more acceptable because it does not involve the creation or destruction of human embryos.
As promising and powerful as they are, current versions of base editors and prime editors can be further optimized and improved. For instance, Cas9 evolved in microorganisms as a nuclease rather than a nickase. Current Cas9 nickases used for base editing (D10A SpCas9 variant) and prime editing (H840A variant) can be engineered to increase their activities and specificities. In parallel, deaminase and reverse transcriptase moieties in base editors and prime editors, respectively, can be engineered or replaced with appropriate orthologues to increase the efficiency and scope of genome editing. It has been shown that base editors can cause both guide RNA-dependent and guide RNA-independent DNA or RNA off-target mutations, raising concerns for their applications in medicine. Prime editors may also cause unwanted on-target and off-target mutations, which must be carefully studied before moving on to therapeutic applications.
Biomedical researchers are now equipped with powerful tools for genome editing. I expect that these tools will be developed further and applied more broadly in both research and medicine in the coming years.
Collins F. The director of the NIH lays out his vision of the future of medical science. Time https://time.com/5709207/medical-science-age-of-discovery (2019).
The National Academies of Sciences, Engineering, and Medicine Organizing Committee for the International Summit on Human Gene Editing. On human gene editing: international summit statement. The National Academies of Sciences, Engineering, and Medicine https://www.nationalacademies.org/news/2015/12/on-human-gene-editing-international-summit-statement (2015).
Centers for Disease Control and Prevention. COVID-19 in racial and ethnic minority groups. CDC https://www.cdc.gov/coronavirus/2019-ncov/need-extra-precautions/racial-ethnic-minorities.html (2020).
Edwards, F., Lee, H. & Esposito, M. Risk of being killed by police use of force in the United States by age, race–ethnicity, and sex. Proc. Natl Acad. Sci. USA 116 , 16793–16798 (2019).
CAS PubMed PubMed Central Google Scholar
Popejoy, A. B. & Fullerton, S. M. Genomics is failing on diversity. Nature 538 , 161–164 (2016).
Popejoy, A. B. et al. The clinical imperative for inclusivity: race, ethnicity, and ancestry (REA) in genomics. Hum. Mutat. 39 , 1713–1720 (2018).
PubMed PubMed Central Google Scholar
Artiga, S. & Orgera, K. Key facts on health and health care by race and ethnicity. Kaiser Family Foundation https://www.kff.org/report-section/key-facts-on-health-and-health-care-by-race-and-ethnicity-coverage-access-to-and-use-of-care/ (2019).
Armstrong, K., Micco, E., Carney, A., Stopfer, J. & Putt, M. Racial differences in the use of BRCA1/2 testing among women with a family history of breast or ovarian cancer. JAMA 293 , 1729–1736 (2005).
CAS PubMed Google Scholar
Bonham, V. L., Callier, S. L. & Royal, C. D. Will precision medicine move us beyond race? N. Engl. J. Med. 374 , 2003–2005 (2016).
Karczewski, K. J. et al. The mutational constraint spectrum quantified from variation in 141,456 humans. Nature 581 , 434–443 (2020).
Khera, A. V. et al. Genome-wide polygenic scores for common diseases identify individuals with risk equivalent to monogenic mutations. Nat. Genet. 50 , 1219–1224 (2018).
The SIGMA Type 2 Diabetes Consortium. Sequence variants in SLC16A11 are a common risk factor for type 2 diabetes in Mexico. Nature 506 , 97–101 (2014).
Google Scholar
Wetterstrand, K. A. DNA sequencing costs: data from the NHGRI genome sequencing program (GSP). National Human Genome Research Institute https://www.genome.gov/sequencingcostsdata (2019).
Wall, J. D. et al. The GenomeAsia 100K Project enables genetic discoveries across Asia. Nature 576 , 106–111 (2019).
CAS Google Scholar
Kowalski, M. H. et al. Use of >100,000 NHLBI Trans-Omics for Precision Medicine (TOPMed) Consortium whole genome sequences improves imputation quality and detection of rare variant associations in admixed African and Hispanic/Latino populations. PLoS Genet. 15 , e1008500 (2019).
Bycroft, C. et al. The UK Biobank resource with deep phenotyping and genomic data. Nature 562 , 203–209 (2018).
Gaziano, J. M. et al. Million veteran program: a mega-biobank to study genetic influences on health and disease. J. Clin. Epidemiol. 70 , 214–223 (2016).
PubMed Google Scholar
Nagai, A. et al. Overview of the BioBank Japan Project: study design and profile. J. Epidemiol. 27 , S2–S8 (2017).
Denny, J. C. et al. Systematic comparison of phenome-wide association study of electronic medical record data and genome-wide association study data. Nat. Biotechnol. 31 , 1102–1110 (2013).
Sirugo, G., Williams, S. M. & Tishkoff, S. A. The missing diversity in human genetic studies. Cell 177 , 1080 (2019).
Martin, A. R. et al. Clinical use of current polygenic risk scores may exacerbate health disparities. Nat. Genet. 51 , 584–591 (2019).
McQuillan, M. A., Zhang, C., Tishkoff, S. A. & Platt, A. The importance of including ethnically diverse populations in studies of quantitative trait evolution. Curr. Opin. Genet. Dev. 62 , 30–35 (2020).
Sohail, M. et al. Polygenic adaptation on height is overestimated due to uncorrected stratification in genome-wide association studies. eLife 8 , e39702 (2019).
Crawford, N. G. et al. Loci associated with skin pigmentation identified in African populations. Science 358 , eaan8433 (2017).
Gasperini, M., Tome, J. M. & Shendure, J. Towards a comprehensive catalogue of validated and target-linked human enhancers. Nat. Rev. Genet. 21 , 292–310 (2020).
Racimo, F., Sankararaman, S., Nielsen, R. & Huerta-Sánchez, E. Evidence for archaic adaptive introgression in humans. Nat. Rev. Genet. 16 , 359–371 (2015).
Skoglund, P. & Mathieson, I. Ancient genomics of modern humans: the first decade. Annu. Rev. Genomics Hum. Genet. 19 , 381–404 (2018).
Vicente, M. & Schlebusch, C. M. African population history: an ancient DNA perspective. Curr. Opin. Genet. Dev. 62 , 8–15 (2020).
Sherman, R. M. et al. Assembly of a pan-genome from deep sequencing of 910 humans of African descent. Nat. Genet. 51 , 30–35 (2019).
Durvasula, A. et al. Recovering signals of ghost archaic introgression in African populations. Sci. Adv. 12 , eaax5097 (2020).
Skov, L. et al. The nature of Neanderthal introgression revealed by 27,566 Icelandic genomes. Nature 582 , 78–83 (2020).
Adeyemo, A. A. et al. ZRANB3 is an African-specific type 2 diabetes locus associated with beta-cell mass and insulin response. Nat. Commun. 10 , 3195 (2019).
Cohen, J. et al. Low LDL cholesterol in individuals of African descent resulting from frequent nonsense mutations in PCSK9 . Nat. Genet. 37 , 161–165 (2005).
Gurdasani, D. et al. Uganda genome resource enables insights into population history and genomic discovery in Africa. Cell 179 , 984–002.e36 (2019).
Gurdasani, D. et al. Genomics of disease risk in globally diverse populations. Nat. Rev. Genet. 20 , 520–535 (2019).
Gulsuner, S. et al. Genetics of schizophrenia in the South African Xhosa. Science 367 , 569–573 (2020).
Shriner, D. & Rotimi, C. N. Whole-genome-sequence-based haplotypes reveal single origin of the sickle allele during the Holocene wet phase. Am. J. Hum. Genet. 102 , 547–556 (2018).
Wu, Y. et al. Highly efficient therapeutic gene editing of human haematopoietic stem cells. Nat. Med. 25 , 776–783 (2019).
Geard, A. et al. Clinical and genetic predictors of renal dysfunctions in sickle cell anaemia in Cameroon. Br. J. Haematol. 178 , 629–639 (2017).
Lebeko, K. et al. Targeted genomic enrichment and massively parallel sequencing identifies novel nonsyndromic hearing impairment pathogenic variants in Cameroonian families. Clin. Genet. 90 , 288–290 (2016).
Genovese, G. et al. Association of trypanolytic ApoL1 variants with kidney disease in African Americans. Science 329 , 841–845 (2010).
Sierra, B. et al. OSBPL10, RXRA and lipid metabolism confer African-ancestry protection against dengue haemorrhagic fever in admixed Cubans. PLoS Pathog. 13 , e1006220 (2017).
Wonkam, A. & de Vries, J. Returning incidental findings in African genomics research. Nat. Genet. 52 , 17–20 (2020).
Provine, W. B. The Origins of Theoretical Population Genetics (University of Chicago Press, 1971)
Fisher, R. A. The correlation between relatives on the supposition of Mendelian inheritance. Trans. R. Soc. Edinb. 52 , 399–433 (1918).
Altenburg, E. & Muller, H. J. The genetic basis of truncate wing – an inconstant and modifiable character in Drosophila. Genetics 5 , 1–59 (1920).
Morton, N. E. Analysis of family resemblance. I. Introduction. Am. J. Hum. Genet. 26 , 318–330 (1974).
Visscher, P. M. et al. 10 Years of GWAS discovery: biology, function and translation. Am. J. Hum. Genet. 101 , 5–22 (2017).
Boyle, E. A., Li, Y. I. & Pritchard, J. K. An expanded view of complex traits: from polygenic to omnigenic. Cell 169 , 1177–1186 (2017).
Emison, E. S. et al. A common, sex-dependent mutation in a putative RET enhancer underlies Hirschsprung disease susceptibility. Nature 434 , 857–863 (2005).
Maurano, M. T. et al. Systematic localization of common disease-associated variation in regulatory DNA. Science 337 , 1190–1195 (2012).
Davidson, E. Emerging properties of animal gene regulatory networks. Nature 468 , 911–920 (2010).
Chatterjee, S. et al. Enhancer variants synergistically drive dysregulation of the RET gene regulatory network in Hirschsprung disease. Cell 167 , 355–368 (2016).
Segal, E., Raveh-Sadka, T., Schroeder, M., Unnerstall, U. & Gaul, U. Predicting expression patterns from regulatory sequence in Drosophila segmentation. Nature 451 , 535–540 (2008).
Chakravarti, A. & Turner, T. N. Revealing rate-limiting steps in complex disease biology: The crucial importance of studying rare, extreme-phenotype families. Bioessays 38 , 578–586 (2016).
Lancaster, M. A. et al. Cerebral organoids model human brain development and microcephaly. Nature 501 , 373–379 (2013).
Rothman, J. & Jarriault, S. Developmental plasticity and cellular reprogramming in caenorhabditis elegans. Genetics 213 , 723–757 (2019).
Porrello, E. R. et al. Transient regenerative potential of the neonatal mouse heart. Science 331 , 1078–1080 (2011).
Mir, M., Bickmore, W., Furlong, E. E. M. & Narlikar, G. Chromatin topology, condensates and gene regulation: shifting paradigms or just a phase? Development 146 , dev182766 (2019).
Ghavi-Helm, Y. et al. Highly rearranged chromosomes reveal uncoupling between genome topology and gene expression. Nat. Genet. 51 , 1272–1282 (2019).
Despang, A. et al. Functional dissection of the Sox9-Kcnj2 locus identifies nonessential and instructive roles of TAD architecture. Nat. Genet. 51 , 1263–1271 (2019).
Hnisz, D., Shrinivas, K., Young, R. A., Chakraborty, A. K. & Sharp, P. A. A phase separation model for transcriptional control. Cell 169 , 13–23 (2017).
Shrinivas, K. et al. Enhancer features that drive formation of transcriptional condensates. Mol. Cell 75 , 549–561 e547 (2019).
Narlikar, G. J. Phase-separation in chromatin organization. J. Biosci. 45 , 5 (2020).
Cao, J. et al. The single-cell transcriptional landscape of mammalian organogenesis. Nature 566 , 496–502 (2019).
Farrell, J. A. et al. Single-cell reconstruction of developmental trajectories during zebrafish embryogenesis. Science 360 , eaar3131 (2018).
Cusanovich, D. A. et al. The cis-regulatory dynamics of embryonic development at single-cell resolution. Nature 555 , 538–542 (2018).
Tang, F. et al. mRNA-Seq whole-transcriptome analysis of a single cell. Nat. Methods 6 , 377–382 (2009).
Camp, J. G., Platt, R. & Treutlein, B. Mapping human cell phenotypes to genotypes with single-cell genomics. Science 365 , 1401–1405 (2019).
Lein, E., Borm, L. E. & Linnarsson, S. The promise of spatial transcriptomics for neuroscience in the era of molecular cell typing. Science 358 , 64–69 (2017).
Satija, R., Farrell, J. A., Gennert, D., Schier, A. F. & Regev, A. Spatial reconstruction of single-cell gene expression data. Nat. Biotechnol. 33 , 495–502 (2015).
Achim, K. et al. High-throughput spatial mapping of single-cell RNA-seq data to tissue of origin. Nat. Biotechnol. 33 , 503–509 (2015).
Cao, J. et al. Comprehensive single-cell transcriptional profiling of a multicellular organism. Science 357 , 661–667 (2017).
Cleary, B., Cong, L., Cheung, A., Lander, E. S. & Regev, A. Efficient generation of transcriptomic profiles by random composite measurements. Cell 171 , 1424–1436 e1418 (2017).
Cao, J. et al. Joint profiling of chromatin accessibility and gene expression in thousands of single cells. Science 361 , 1380–1385 (2018).
Kester, L. & van Oudenaarden, A. Single-cell transcriptomics meets lineage tracing. Cell Stem Cell 23 , 166–179 (2018).
Stuart, T. & Satija, R. Integrative single-cell analysis. Nat. Rev. Genet. 20 , 257–272 (2019).
Zhu, C., Preissl, S. & Ren, B. Single-cell multimodal omics: the power of many. Nat. Methods 17 , 11–14 (2020).
Anzalone, A. V., Koblan, L. W. & Liu, D. R. Genome editing with CRISPR-Cas nucleases, base editors, transposases and prime editors. Nat. Biotechnol . (2020).
Loeffler, D. et al. Asymmetric lysosome inheritance predicts activation of haematopoietic stem cells. Nature 573 , 426–429 (2019).
Schmidt, F., Cherepkova, M. Y. & Platt, R. J. Transcriptional recording by CRISPR spacer acquisition from RNA. Nature 562 , 380–385 (2018).
Holliday, R. & Pugh, J. E. DNA modification mechanisms and gene activity during development. Science 187 , 226–232 (1975).
Strahl, B. D. & Allis, C. D. The language of covalent histone modifications. Nature 403 , 41–45 (2000).
Jambhekar, A., Dhall, A. & Shi, Y. Roles and regulation of histone methylation in animal development. Nat. Rev. Mol. Cell Biol. 20 , 625–641 (2019).
Smith, Z. D. et al. Epigenetic restriction of extraembryonic lineages mirrors the somatic transition to cancer. Nature 549 , 543–547 (2017).
Jinek, M. et al. A programmable dual-RNA-guided DNA endonuclease in adaptive bacterial immunity. Science 337 , 816–821 (2012).
Nabet, B. et al. The dTAG system for immediate and target-specific protein degradation. Nat. Chem. Biol. 14 , 431–441 (2018).
Clevers, H. Modeling development and disease with organoids. Cell 165 , 1586–1597 (2016).
Dekker, J., Marti-Renom, M. A. & Mirny, L. A. Exploring the three-dimensional organization of genomes: interpreting chromatin interaction data. Nat. Rev. Genet. 14 , 390–403 (2013).
Banani, S. F., Lee, H. O., Hyman, A. A. & Rosen, M. K. Biomolecular condensates: organizers of cellular biochemistry. Nat. Rev. Mol. Cell Biol. 18 , 285–298 (2017).
Lupianez, D. G. et al. Disruptions of topological chromatin domains cause pathogenic rewiring of gene-enhancer interactions. Cell 161 , 1012–1025 (2015).
Basu, S. et al. Unblending of transcriptional condensates in human repeat expansion disease. Cell 181 , 1062–1079 e1030 (2020).
Grosswendt, S. et al. Epigenetic regulator function through mouse gastrulation. Nature 584 , 102–108 (2020).
Johnson, T. B. & Coghill, R. D. Researches on pyrimidines. C111. The discovery of 5-methyl-cytosine in tuberculinic acid, the nucleic acid of the tubercle bacillus. J. Am. Chem. Soc. 47 , 2838–2844,47 (1925).
Heard, E. et al. Ten years of genetics and genomics: what have we achieved and where are we heading? Nat. Rev. Genet. 11 , 723–733 (2010).
Quinn, J. J. & Chang, H. Y. Unique features of long non-coding RNA biogenesis and function. Nat. Rev. Genet. 17 , 47–62 (2016).
Kopp, F. & Mendell, J. T. Functional classification and experimental dissection of long noncoding RNAs. Cell 172 , 393–407 (2018).
Liu, S. J. et al. CRISPRi-based genome-scale identification of functional long noncoding RNA loci in human cells. Science 355 , eaah7111 (2017).
Rubin, A. J. et al. Coupled single-cell CRISPR screening and epigenomic profiling reveals causal gene regulatory networks. Cell 176 , 361–376.e17 (2019).
Quinn, J. J. et al. Rapid evolutionary turnover underlies conserved lncRNA-genome interactions. Genes. Dev. 30 , 191–207 (2016).
Kirk, J. M. et al. Functional classification of long non-coding RNAs by k-mer content. Nat. Genet. 50 , 1474–1482 (2018).
Carter, A. C. et al. Spen links RNA-mediated endogenous retrovirus silencing and X chromosome inactivation. eLife 9 , e54508 (2020).
Lubelsky, Y. & Ulitsky, I. Sequences enriched in Alu repeats drive nuclear localization of long RNAs in human cells. Nature 555 , 107–111 (2018).
Shukla, C. J. et al. High-throughput identification of RNA nuclear enrichment sequences. EMBO J. 37 , e98452 (2018).
Czerminski, J. T. & Lawrence, J. B. Silencing Trisomy 21 with XIST in neural stem cells promotes neuronal differentiation. Dev. Cell 52 , 294–308 e3 (2020).
Martínez-Jiménez, F. et al. A compendium of mutational cancer driver genes. Nat. Rev. Cancer https://doi.org/10.1038/s41568-020-0290-x (2020).
Article PubMed Google Scholar
Wilkinson, M. et al. The FAIR guiding principles for scientific data management and stewardship. Sci. Data 3 , 160018 (2016).
Alexandrov, L. B. et al. The repertoire of mutational signatures in human cancer. Nature 578 , 94–101 (2020).
Gonzalez-Perez, A., Radhakrishnan, S. & Lopez-Bigas, N. Local determinants of the mutational landscape of the human genome. Cell 177 , 101–114 (2019).
Martincorena, I. et al. High burden and pervasive positive selection of somatic mutations in normal human skin. Science 348 , 880–886 (2015).
Martincorena, I. et al. Somatic mutant clones colonize the human esophagus with age. Science 362 , 911–917 (2018).
Yokoyama, A. et al. Age-related remodelling of oesophageal epithelia by mutated cancer drivers. Nature 565 , 312–317 (2019).
Genovese, G. et al. Clonal hematopoiesis and blood-cancer risk inferred from blood DNA sequence. N. Engl. J. Med. 371 , 2477–2487 (2014).
Jaiswal, S. et al. Age related clonal hematopoiesis associated with adverse outcomes. N. Engl. J. Med. 371 , 2488–2498 (2014).
Sabarinathan, R. et al. The whole-genome panorama of cancer drivers. Preprint at bioRxiv https://doi.org/10.1101/190330 (2017).
Pich, O. et al. The mutational footprints of cancer therapies. Nat. Genet. 51 , 1732–1740 (2019).
Campbell, P. J. et al. Pan-cancer analysis of whole genomes. Nature 578 , 82–93 (2020).
Rozenblatt-Rosen, O., Stubbington, M. J. T., Regev, A. & Teichmann, S. A. The Human Cell Atlas: from vision to reality. Nature 550 , 451–453 (2017).
ENCODE Project Consortium. An integrated encyclopedia of DNA elements in the human genome. Nature 489 , 57–74 (2012).
Damodaran, S. et al. Cancer Driver Log (CanDL): catalog of potentially actionable cancer mutations. J. Mol. Diagn. 17 , 554–559 (2015).
High, K. A. & Roncarolo, M. G. Gene therapy. N. Engl. J. Med. 381 , 455–464 (2019).
Shilo, S., Rossman, H. & Segal, E. Axes of a revolution: challenges and promises of big data in healthcare. Nat. Med. 26 , 29–38 (2020).
Kotler, E. et al. A systematic p53 mutation library links differential functional impact to cancer mutation pattern and evolutionary conservation. Mol. Cell 71 , 873 (2018).
Swanson, J. M. The UK Biobank and selection bias. Lancet 380 , 110 (2012).
Maini Rekdal, V., Bess, E. N., Bisanz, J. E., Turnbaugh, P. J. & Balskus, E. P. Discovery and inhibition of an interspecies gut bacterial pathway for levodopa metabolism. Science 364 , eaau6323 (2019).
Hernán, M. A. & Robins, J. M. Using big data to emulate a target trial when a randomized trial is not available. Am. J. Epidemiol. 183 , 758–764 (2016).
Nelson, M. R. et al. The support of human genetic evidence for approved drug indications. Nat. Genet. 47 , 856–860 (2015).
Kim, J.-S. Genome editing comes of age. Nat. Protoc. 11 , 1573–1578 (2016).
Kim, D. et al. Digenome-seq: genome-wide profiling of CRISPR-Cas9 off-target effects in human cells. Nat. Methods 12 , 237–243 (2015).
Tsai, S. Q. et al. GUIDE-seq enables genome-wide profiling of off-target cleavage by CRISPR-Cas nucleases. Nat. Biotechnol. 33 , 187–197 (2015).
Wienert, B. et al. Unbiased detection of CRISPR off-targets in vivo using DISCOVER-Seq. Science 364 , 286–289 (2019).
Kosicki, M., Tomberg, K. & Bradley, A. et al. Repair of double-strand breaks induced by CRISPR-Cas9 leads to large deletions and complex rearrangements. Nat. Biotechnol. 36 , 765–771 (2018).
Komor, A. C. et al. Programmable editing of a target base in genomic DNA without double-stranded DNA cleavage. Nature 533 , 420–424 (2016).
Nishida, K. et al. Targeted nucleotide editing using hybrid prokaryotic and vertebrate adaptive immune systems. Science 353 , aaf8729 (2016).
Anzalone, A. V. et al. Search-and-replace genome editing without double-strand breaks or donor DNA. Nature 576 , 149–157 (2019).
Ma, H. et al. Correction of a pathogenic gene mutation in human embryos. Nature 548 , 413–419 (2017).
Download references
Acknowledgements
A.C. acknowledges that the ideas in his contribution were developed through studies on Hirschsprung disease and thanks the many trainees who have contributed to this work over the past 5 years. A.L.M. acknowledges A. Gutierrez, K. Kostick, G. Lazaro, M. Majumder, K. Munoz, S. Pereira, H. Smith and P. Zuk for feedback. A.M. thanks D. Hnisz, Z. D. Smith, J. Charlton and H. Kretzmer for feedback and the Max Planck Society for funding. A.W. is supported by NIH awards U54HG009790, U01HG009716, U01HG007459 and U24HL135600, and Wellcome Trust award H3A/18/001, and states that the funders had no role in study design, and analysis, decision to publish or preparation of the manuscript. B.T. acknowledges J. G. Camp for helpful discussions. E.E.M.F. is very grateful to A. Ephrussi, M. Mir, M. Perino, Y. Kherdjemil, T. Pollex and S. Secchia for useful comments. E. E. M. F is supported by European Research Council (Advanced Grant) agreement no. 787611 (DeCRyPT). E.S. is supported by grants from the European Research Council and the Israel Science Foundation. H.Y.C. is supported by NIH RM1-HG007735 and R35-CA209919. H.Y.C. is an investigator of the Howard Hughes Medical Institute. J.-S.K. is supported by the Institute for Basic Science (IBS-R021-D1). N.L-B. acknowledges funding from the European Research Council (Consolidator Grant 682398), the Spanish Ministry of Economy and Competitiveness (SAF2015-66084-R, European Regional Development Fund) and the Asociación Española Contra el Cáncer (GC16173697BIGA). S.A.T. is funded by NIH grants R35 GM134957-01 and NIAMS R01AR076241-01A1 and American Diabetes Association Pathway to Stop Diabetes grant #1-19-VSN-02.
Author information
Authors and affiliations.
Center for Medical Ethics and Health Policy, Baylor College of Medicine, Houston, TX, USA
Amy L. McGuire
Broad Institute of MIT and Harvard, Cambridge, MA, USA
Stacey Gabriel & Alexander Meissner
Department of Genetics, University of Pennsylvania, Philadelphia, PA, USA
Sarah A. Tishkoff
Department of Biology, University of Pennsylvania, Philadelphia, PA, USA
Department of Medicine, Faculty of Health Sciences, University of Cape Town, Cape Town, South Africa
Ambroise Wonkam
Institute of Infectious Diseases and Molecular Medicine, Faculty of Health Sciences, University of Cape Town, Cape Town, South Africa
Center for Human Genetics and Genomics, New York University Grossman School of Medicine, New York, NY, USA
Aravinda Chakravarti
European Molecular Biology Laboratory, Genome Biology Department, Heidelberg, Germany
Eileen E. M. Furlong
Department of Biosystems Science and Engineering, ETH Zurich, Basel, Switzerland
Barbara Treutlein
Department of Genome Regulation, Max Planck Institute for Molecular Genetics, Berlin, Germany
Alexander Meissner
Department of Stem Cell and Regenerative Biology, Harvard University, Cambridge, MA, USA
Institute of Chemistry and Biochemistry, Freie Universität Berlin, Berlin, Germany
Center for Personal Dynamic Regulomes, Howard Hughes Medical Institute, Stanford University, Stanford, CA, USA
Howard Y. Chang
Institute for Research in Biomedicine (IRB Barcelona), The Barcelona Institute of Science and Technology, Barcelona, Spain
Núria López-Bigas
Research Program on Biomedical Informatics, Universitat Pompeu Fabra, Barcelona, Spain
Institució Catalana de Recerca i Estudis Avançats, Barcelona, Spain
Department of Computer Science and Applied Mathematics, Weizmann Institute of Science, Rehovot, Israel
Center for Genome Engineering, Institute for Basic Science, Daejon, Republic of Korea
Jin-Soo Kim
You can also search for this author in PubMed Google Scholar
Corresponding authors
Correspondence to Amy L. McGuire , Stacey Gabriel , Sarah A. Tishkoff , Ambroise Wonkam , Aravinda Chakravarti , Eileen E. M. Furlong , Barbara Treutlein , Alexander Meissner , Howard Y. Chang , Núria López-Bigas , Eran Segal or Jin-Soo Kim .
Ethics declarations
Competing interests.
H.Y.C. is a co-founder of Accent Therapeutics and Boundless Bio and an advisor of 10x Genomics, Arsenal Biosciences and Spring Discovery. J.-S.K. is a co-founder of and holds stock in ToolGen Inc. A.C., A.L.M., A.M., A.W., B.T., E.E.M.F., E.S., N.L.-B., S.G. and S.A.T. declare no competing interests.
Additional information
Publisher’s note.
Springer Nature remains neutral with regard to jurisdictional claims in published maps and institutional affiliations.
Related links
Beyond 1 Million Genomes : https://b1mg-project.eu/
Blueprint Epigenome : https://www.blueprint-epigenome.eu/
cBioPortal for Cancer Genomics : https://www.cbioportal.org/
ENCODE : https://www.encodeproject.org/
Global Alliance for Genomics and Health : https://www.ga4gh.org/
gnomAD : https://gnomad.broadinstitute.org/
GTEx : https://www.gtexportal.org/home/
GWAS Catalog : https://www.ebi.ac.uk/gwas
H3Africa : https://h3africa.org
Roadmap Epigenomics Project : http://www.roadmapepigenomics.org/
Rights and permissions
Reprints and permissions
About this article
Cite this article.
McGuire, A.L., Gabriel, S., Tishkoff, S.A. et al. The road ahead in genetics and genomics. Nat Rev Genet 21 , 581–596 (2020). https://doi.org/10.1038/s41576-020-0272-6
Download citation
Accepted : 21 July 2020
Published : 24 August 2020
Issue Date : October 2020
DOI : https://doi.org/10.1038/s41576-020-0272-6
Share this article
Anyone you share the following link with will be able to read this content:
Sorry, a shareable link is not currently available for this article.
Provided by the Springer Nature SharedIt content-sharing initiative
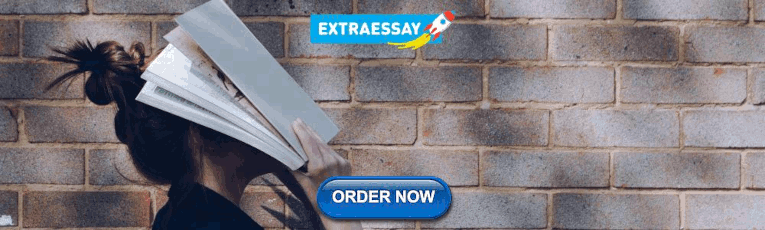
Quick links
- Explore articles by subject
- Guide to authors
- Editorial policies
Sign up for the Nature Briefing: Translational Research newsletter — top stories in biotechnology, drug discovery and pharma.

- Undergraduate
- High School
- Architecture
- American History
- Asian History
- Antique Literature
- American Literature
- Asian Literature
- Classic English Literature
- World Literature
- Creative Writing
- Linguistics
- Criminal Justice
- Legal Issues
- Anthropology
- Archaeology
- Political Science
- World Affairs
- African-American Studies
- East European Studies
- Latin-American Studies
- Native-American Studies
- West European Studies
- Family and Consumer Science
- Social Issues
- Women and Gender Studies
- Social Work
- Natural Sciences
- Pharmacology
- Earth science
- Agriculture
- Agricultural Studies
- Computer Science
- IT Management
- Mathematics
- Investments
- Engineering and Technology
- Engineering
- Aeronautics
- Medicine and Health
- Alternative Medicine
- Communications and Media
- Advertising
- Communication Strategies
- Public Relations
- Educational Theories
- Teacher's Career
- Chicago/Turabian
- Company Analysis
- Education Theories
- Shakespeare
- Canadian Studies
- Food Safety
- Relation of Global Warming and Extreme Weather Condition
- Movie Review
- Admission Essay
- Annotated Bibliography
- Application Essay
- Article Critique
- Article Review
- Article Writing
- Book Review
- Business Plan
- Business Proposal
- Capstone Project
- Cover Letter
- Creative Essay
- Dissertation
- Dissertation - Abstract
- Dissertation - Conclusion
- Dissertation - Discussion
- Dissertation - Hypothesis
- Dissertation - Introduction
- Dissertation - Literature
- Dissertation - Methodology
- Dissertation - Results
- GCSE Coursework
- Grant Proposal
- Marketing Plan
- Multiple Choice Quiz
- Personal Statement
- Power Point Presentation
- Power Point Presentation With Speaker Notes
- Questionnaire
- Reaction Paper
- Research Paper
- Research Proposal
- SWOT analysis
- Thesis Paper
- Online Quiz
Literature Review
- Movie Analysis
- Statistics problem
- Math Problem
- All papers examples
- How It Works
- Money Back Policy
- Terms of Use
- Privacy Policy
- We Are Hiring
Genetic Testing, Essay Example
Pages: 5
Words: 1244
Hire a Writer for Custom Essay
Use 10% Off Discount: "custom10" in 1 Click 👇
You are free to use it as an inspiration or a source for your own work.
Genetic testing also known as DNA-based testing involves examining DNA molecules to find possible signs of genetic disorders. The advancements in the field of genetics have rapidly pushed the boundaries of medical science and have made it possible to predict the probability of genetic disorders to occur in the individuals. Thus, the greatest promise of genetic testing is not only in preventive measures but further advancements in genetics are expected to produce techniques that may even be able to repair faulty genes. Currently, there are more than 1000 genetic tests available from the testing laboratories including Alzheimer’s disease, Cystic fibrosis, Huntington’s disease, Sickle cell disease, and Timothy Syndrome.
As with any disruptive technology, the genetic testing raises certain ethical and moral issues. Privacy is one of the major concerns because genetic testing results could be used by insurance companies and other commercial enterprises to decide whether to provide their services or not and may charge more to the customers they perceive as high-risk. In addition, genetic testing is not fool-proof and the misleading results may lead to inaccurate treatments and preventive measures. In addition, the technology is still in infancy and any information that can’t be interpreted with reliability even if it’s accurate is as useless as no information at all. The medical community has no professional standards or guidelines that could be used to analyze the genetic testing results which results in doctors applying inconsistent analytical tools and reaching inconsistent conclusions.
The results of the genetic testing may inflict emotional pain on the individuals even if they are inaccurate. The psychosocial risks may be guilt, anxiety, impaired self-esteem, social stigma, and employment discrimination (American Academy of Pediatrics). There may be financial risks if the customer decides to act on the information and opt for expensive medical treatments in hope of reducing the risks indicated by genetic test. Moreover, genetic information has limited predictive power as our genes interact with the environment in complex ways.
As far as genetic testing in pediatrics is concerned, the American Academy of Pediatrics recommends genetic testing only when it is in the best interests of the child and when the legitimate interests of the parent and the family can be promoted without anticipated harm to the child. It has been argued that genetic testing for children should be mandatory because a society has an obligation to promote child welfare through detective and timely treatment of selected conditions. At the same time, parents have a tendency to underestimate the risks involved in treatments on the basis of genetic test which may not promote the best interests of the child (American Academy of Pediatrics).
Emory Law Journal provides an interesting hypothesis on the potential impact of media on consumer choices. American actress Christina Applegate appeared on the Oprah Winfrey show on September 30, 2008 and declared that her decision to remove both of her breasts was based on her genetic test. She remarked, “I’m clear. Absolutely 100 percent clear and clean.” This information could be misinterpreted by the female viewers who have a family history of breast cancer. They may order their genetic test and decide to go the Christina Applegate way. But Christina Applegate’s self-assurance was not exactly correct because double mastectomy significantly decreases the chance of later developing breast cancer but does not guarantee prevention. In addition, direct-to-consumer companies have no obligation to tell customers of the treatment choices available and the customers may underestimate the social and emotional distress that breast removal may cause them later. Direct-to-consumer companies have a potential to mislead customers because even though they issue disclaimer that their results cannot be used to make medical decisions and that the users assume all the risk, their marketing messages send hope and promise of healthy future. Genetic testing companies are avoiding the possibility of legal problems by masking themselves as seller of informational and recreational services (Kishore, 2010).
The pace of regulations to govern the trade practices of the direct-to-consumer companies may have yet to come but the issue has not escaped the attention of the government. United States Government Accountability Office (GAO) tested direct-to-consumer genetic testing companies and found that they made medically unproven claims. In addition, the results from all the four companies whose services GAO purchased yielded results that were inconsistent with each other and the companies didn’t inform of their inability to carry out DNA tests on races prior to the purchase. In addition, the individual companies yielded different test results on the two samples that were actually the same. Some companies even tried to sell supplements that were supposed to repair damaged DNAs. In addition, they used fraudulent endorsements from high profile athletes (Kutz). This shows that genetic testing is still unreliable and a huge risk exists in utilizing genetic tests for making important medical decisions.
There is also a risk that genetic tests may be abused by employers to predict the probability of undesirable behavior in individuals which may or may never happen. For example, if an individual possesses a gene variant which studies link to increase risk of substance abuse such as alcohol and drugs, the employer may decide he doesn’t want to hire a potential future liability (Bailey).
Genetic testing may have limited useful and reliable applications especially in the case of diseases whose genes are few and have been correctly identified. Huntington gene is one example. People with Huntington disease have 36 to more than 120 CAG (Huntington disease is also known as CAG trinucleotide repeat expansion). People with 36 to 40 CAG repeats may or may not develop the signs of Huntington disease but people with more than 40 repeats almost always develop the disorder (Genetics Home Reference).
Genetic testing if proved negative may give false hopes to the customers. Customers may become careless with their life habits and may even forego regular diagnosis tests later in life. Thus, just as positive results may result in over reaction, negative results may lead to carelessness on the part of the customers. Genetic tests point towards a bright future of medical science as further progress is made but it will take some time for genetic testing to become a truly reliable medical service. Even when genetic testing is taken, the importance of medical advice should not be underestimated. Medical professionals are better informed due to their experience and knowledge and are better aware of the various options available to the customers.
Genetic testing may have consequences that extend far beyond the individuals. Genetic testing may persuade couples to opt for abortion or totally forego procreation plans. Some people may object on the basis of their moral values that humans are trying to imitate God which could limit federal funding to fund genetic research and slow down the progress in genetics. This has already happened when President Bill Clinton sent bill to the Congress to outlaw the cloning of humans on the recommendation of the National Bioethics Advisory Commission (Human Genome News, 1997).
American Academy of Pediatrics. Ethical Issues With Genetic Testing in Pediatrics. 3 February 2011 <http://aappolicy.aappublications.org/cgi/content/full/pediatrics;107/6/1451>.
Bailey, Ronald. “I’ll Show You My Genome. Will You Show Me Yours?” Reason January 2011: 35-43.
Genetics Home Reference. HTT. October 2008. 3 February 2011 <http://ghr.nlm.nih.gov/gene/HTT>.
Human Genome News. President’s Bill Would Prohibit Human Cloning. January-June 1997. 3 February 2011 <http://www.ornl.gov/sci/techresources/Human_Genome/publicat/hgn/v8n3/07pres.shtml>.
Kishore, Deepthy. “Test at Your Own Risk: Your Genetic Report Card and the Direct-To-Consumer Duty to Secure Informed Consent.” Emory Law Journal 2010: 1553-1609.
Kutz, Gregory. “Direct-To-Consumer Genetic Tests: Misleading Test Results Are Further Complicated by Deceptive Marketing and Other Questionable Practices.” Investigative. 2010.
Stuck with your Essay?
Get in touch with one of our experts for instant help!
The Language of Attraction, Essay Example
Federal Budget, Literature Review Example
Time is precious
don’t waste it!
Plagiarism-free guarantee
Privacy guarantee
Secure checkout
Money back guarantee
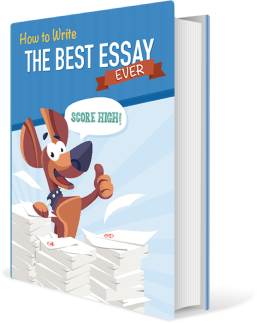
Related Essay Samples & Examples
Voting as a civic responsibility, essay example.
Pages: 1
Words: 287
Utilitarianism and Its Applications, Essay Example
Words: 356
The Age-Related Changes of the Older Person, Essay Example
Pages: 2
Words: 448
The Problems ESOL Teachers Face, Essay Example
Pages: 8
Words: 2293
Should English Be the Primary Language? Essay Example
Pages: 4
Words: 999
The Term “Social Construction of Reality”, Essay Example
Words: 371
- Future Students
- Current Students
- Faculty and Staff
- Corporate Partners
- Request Info
- Quick Links
Why is it Important to Study Genetics?
September 20, 2023
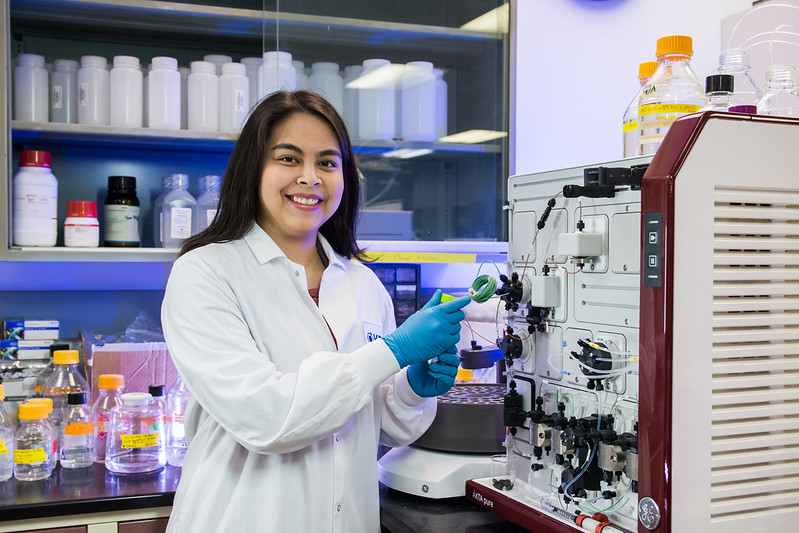
Studying genetics and how it affects people’s lives is crucial to advancing science across a multitude of disciplines. Genetics is now intrinsic to modern biology, and genetic breakthroughs have impacted everything from our understanding of diseases and their treatment to the foods we eat and the clothes we wear every day. But why is it important to study genetics? Let’s explore the reasons why.
4 Reasons Why the Study of Genetics is Important
1. studying genetics gives an understanding of where we’ve been .
Studying genetics and its history provides the foundation for all future advancements. Knowing where genetic science has come from and the hurdles overcome by research helps students find their place in the greater story of genes. Having a picture of what research already exists can also help scientists connect old research and new discoveries, especially as technology advances.
2. Studying Genetics Shows Us Where We Can Go
When we understand what advances have already come from genetic science, it’s easier to think up new technologies and processes to do the most good. From integrating new technology to making groundbreaking discoveries, taking the time to get rooted in the science behind genetics is the first step toward greatness.
3. Studying Genetics Changes the Lives of People Waiting for a Diagnosis
Genes give answers for what’s happening in our bodies, from disabilities to diseases like cancer and heart disease. Understanding the genetic qualities of a person helps scientists and doctors understand how to best treat them and gives patients more peace of mind. Genetics plays a big role in diagnosis, providing answers — faster — for people seeking treatment. And when patients are misdiagnosed, genetic research can help them receive the correct diagnosis and start the right course of treatment.
4. Studying Genetics Helps to Customize Medicine
Did you know that people respond differently to various medications and treatments based on their genetic makeup? It’s true—and this not only helps personalize medicine and treatment but can also lead to more effective treatments with fewer side effects.
Change Diagnosis Stories for Generations: Study Genetics at KGI
The Master of Science in Human Genetics and Genetic Counseling (MSGC) program at Keck Graduate Institute (KGI) in Claremont, CA, is dedicated to educating innovative genetic counselors who will serve the needs of individual patients, the healthcare system, and the bioscience industry.
Our program emphasizes interprofessional collaboration, systematic problem-solving, the safe, efficient, and ethical use of technology and biotechnology, and personalized patient care. It prepares graduates to be leaders among healthcare professionals and be dedicated to delivering advanced, personally-optimized patient care. Through genetic counseling, society benefits. And at KGI, we’re leading advancements in the field.
To learn more about what you can achieve when you study genetics, download our guide, There’s More to Medicine Than Meets the Eye: Your Guide to a Career in Healthcare and the Life Sciences . In it, you’ll get a closer look at KGI’s celebrated programs and where a degree in genetics can take you.
Or, you can request more information about our programs. Ready to start your journey into genomic science today? Apply to KGI !
View All Events
What Are Some Popular Careers in ...
What is genetic counseling.
Connect with us to hear how you can use your passions in STEM to impact patients’ lives & accelerate healthcare innovation. Fill out the form & we’ll be in touch with more information!
Explore our Additional Resources
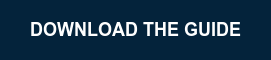
REVIEW article
A systematic review of the psychological implications of genetic testing: a comparative analysis among cardiovascular, neurodegenerative and cancer diseases.
- 1 Department of Oncology and Hematoncology, Interdisciplinary Research Center on Decision Making Processes, University of Milan, Milan, Italy
- 2 Applied Research Division for Cognitive and Psychological Science, IEO Istituto Europeo di Oncologia, Milan, Italy
Background: Genetic testing is performed for different purposes, such as identifying carriers, predicting a disease onset in presymptomatic individuals or confirming a diagnosis. However, these tests may have notable psychological effects, such as generating anxiety and depression. These effects may depend on people's perception of risk, severity, and controllability of the disease; and the availability of treatments. To date, there are no reports that analyze these factors specifically, and their role in influencing genetic test users' experience.
Methods: We performed a systematic review of the psychological implication of undergoing genetic testing for cardiovascular, neurodegenerative and cancer diseases. Articles were searched on PubMed, Google Scholar, and PsychInfo.
Results: 47 studies were included, 9 concerning cardiovascular disease, 18 neurodegenerative disorders, and 20 for cancer disease. According to the reviewed studies, people experience no significant increase in distress and anxiety, or adverse impacts on quality of life, except the Huntington disease, which is characterized by depressive symptoms, suicidal ideations, and hopelessness in gene carriers. People tend to consider genetic tests as valid information to take important preventive decisions. Genetic risk for cardiovascular disease is perceived to be manageable; genetic analysis for some neurodegenerative diseases (e.g., Alzheimer) or cancer (breast cancer in particular) is considered useful because the problem could be addressed in advance with preventive behaviors.
Conclusions: Genetic tests should be proposed along with proper psychological support and counseling focused on users' genetic health literacy; perception of risk, beliefs about disease controllability, in order to foster fruitful medical decisions.
Introduction
Cancer, cardiovascular diseases and dementia are among the main causes of mortality and morbidity in Europe. Since they will have even larger economic implications in the future, policy-makers have increasingly focused their attention on them ( Abegunde et al., 2007 ; Désesquelles et al., 2014 ; Mackenbach et al., 2014 ; Suzman et al., 2015 ). These conditions affect many people worldwide often causing an impairment of the quality of life and psychosocial well-being, thus they require the attention of the scientific community. In 2015 cardiovascular disease death were 17.92 million, with 422.7 million of cases worldwide ( Roth et al., 2017 ). The WHO estimated that 17.7 million people died from cardiovascular disease last year. Alzheimer disease (AD) affects approximately 24 million people globally ( Erkkinen et al., 2018 ) and this number could quadruple by 2050. Currently, dementia is reported to be the leading cause of mortality in England and Wales (Office for National Statistics ONS), and in 2015 the Eurostat (the Directorate-General of the European Commission) reported 213,000 deaths in Europe caused by nervous system diseases including Alzheimer's.
Regarding cancer data, 14.1 million new cancer cases and 8.2 million cancer deaths occurred in 2012 worldwide ( Ferlay et al., 2015 ), which in 2015 grew to 8.8 million (WHO).
These complex conditions usually need long-term treatments and care, involving different health professionals, expensive drugs, access to medical equipment, putting a large burden on society.
The growing diagnoses of many chronic diseases are associated with an aging population, but also with lifestyle choices such as smoking, diet and exercise, and genetic predisposition ( WHO and FAO, 2003 ; World Health Organisation, 2014 ).
In the last decades, there have been considerable investments in genomics (DNA-based) research to study susceptibility to cancer and other chronic diseases and to promote new preventive interventions ( Walter and Emery, 2012 ). Currently, the use of family health history and multiplex genetic tests to identify an individual's risk for multiple diseases simultaneously is a frequent clinical practice ( Yang et al., 2003 ; Yoon and Scheuner, 2003 ; Khoury et al., 2004 ; Yoon, 2005 ). Many associations between single-nucleotide polymorphisms (SNPs) and risks for common complex diseases have been identified. Genetic testing generally provides information about the presence of these genetic variants (SNPs), which could represent an increased risk of developing the disease. Their clinical utility depends on how much the knowledge about this genetic variant could give additional information concerning diagnosis, prognosis or contribute to disease management. Available types of testing include for instance diagnostic, carrier, predictive and susceptibility tests. Diagnostic tests confirm a diagnosis when a particular condition is suspected, based on physical symptoms. Carrier testing identifies people who carry one copy of a gene mutation that can be inherited by their offspring. Predictive and susceptibility testing identifies mutations that increase a person's risk of developing disorders with a genetic basis. These tests may help people making decisions about their daily habits or medical care. For instance, discovering the susceptibility for breast cancer, or stroke could allow people to change their lifestyle, nutrition and “take steps to reduce those risks for which interventions are or will be available” ( Collins and McKusick, 2001 ). Nonetheless, not all kinds of genetic testing are useful for clinical management or outcomes improvement, either because of a lack of treatments, uselessness for the personal decision, or absence of scientific evidence for the genetic predisposition. Proven clinical utility and cost-effectiveness need to be carefully evaluated when considering the implementation of genetic testing in healthcare ( Cornel et al., 2014 ), even when considering the recent discoveries which have underlined the heterogeneity of chronic diseases and the importance of gene-environment interaction in modulating disease onset and responses to preventive interventions ( Curtis et al., 2012 ).
In spite of these premises, a recent study, published by the Market Research Future (the “Global Genetic Testing Market - Forecasts from 2018 to 2023” see https://www.marketresearchfuture.com/ ), reported the amount of genetic tests performed in the European and non-European countries based on the subdivision of pathologies or on the type of genetic test (diagnostic, predictive, etc.). The study estimated a global growth of genetic testing market at a Compounded Average Growth Rate (CAGR) of 12.94% by 2023. This exponential growth should go hand in hand with an appropriate genetic counseling practice, but to date genetic information is often given to people with poor genetic literacy without a specific psychological assessment ( Burke et al., 2002 ) or genetic testing users do not receive a specific pre and post-test counseling ( Janssens et al., 2017 ). For instance, in Italy, only 12% of all genetic analyses had been accompanied by pre or post-test counseling ( Giardino et al., 2016 ).
The genetic counseling is usually provided by trained professionals, mainly geneticists, who explain the genetic aspects of illnesses and the risk of developing or passing an illness to their offspring (WHO). It should address patients' concerns, and one of their families, to help with the decision-making process. Nevertheless, this not always happened in recent years especially with the introduction of direct to consumers genetic testing (DTC), genetic tests sold to people without a medical intermediate ( Oliveri and Pravettoni, 2016 ).
Starting from these premises, we could infer that genetic risk communication might deeply affect people's lives and habits. To date, studies on the psychological impact of genetic tests mainly focused on “harmful” reactions, such as anxiety, distress, and depression, when receiving genetic risk information, obtaining discordant results and without any explanation/discussion for this discrepancy ( Oliveri et al., 2016a ). Previous reviews revealed that DNA based disease risk has little or no effect on health-related behaviors ( Heshka et al., 2008 ; Hollands et al., 2016 ). We should consider that genetic testing impact also depends on how people perceive their risk, severity, and controllability related to specific categories of disease ( Cameron and Muller, 2009 ; Wang et al., 2009 ; Wade et al., 2012 ); on the genetic tests predictability or nature of the diseases (from monogenic to genetic susceptibility factors), and on the presence/absence of treatments ( Cameron and Muller, 2009 ).
People's emotional reactions to genetic testing and how risk perceptions vary from diseases to disease are fundamental aspects to be investigated on, in order to correlate preventive behaviors they may, or may not, adopt ( DiLorenzo et al., 2006 ; Shiloh et al., 2013 ).
For this reason, we aim to provide a comprehensive overview of studies realized in the last two decades (2000–2016), which investigated psychological and behavioral issues after having undergone genetic testing for different categories of chronic diseases. The purpose is to identify a limited number of overarching psychological reactions for each condition. In particular, we chose to compare neurodegenerative, cardiovascular and cancer diseases, because of their differences in treatments availability and preventive options (e.g., there are fewer preventive options for neurodegenerative disorders compared to cancer or cardiovascular diseases, where risk is in some cases manageable with screenings or healthier lifestyles). Moreover, people have different beliefs and perception of the controllability for these diseases which could affect their reaction to a positive genetic test result.
Study Design and Search Strategy
Potential eligible articles were systematically searched on PubMed, Google Scholar and PsychInfo using the following combinations of terms: “psychological outcomes,” “psychological impact,” “genetic test,” “genetic risk,” “neurodegenerative disorders,” “cancer,” and “cardiovascular disease.” Depending on the disease for which the genetic test was performed, we allocated the collected articles into three general categories: Cancer (C), Cardiovascular diseases (CV), and Neurodegenerative disorders (N) (see Table 1 ). Following criteria were considered to include articles:
(a) studies in which a psycho-behavioral and/or quality of life evaluation after having received genetic test results was performed;
(b) studies in which subjects tested were adults.

Table 1 . Characteristics of studies evaluating psychological impact of genetic testing for cardiovascular, neurodegenerative and cancer diseases.
Exclusion criteria were determined by the aim to analyze the “raw” impact genetic test information can have on people's psychological reactions and/or quality of life, without the mediation of interventions (e.g., counseling), or specific population (e.g., children) or phenomenon (e.g., Direct to Consumer genetic testing).
Exclusion criteria were as follow:
(a) investigation of prenatal screening, or childhood and adolescent genetic testing;
(b) investigation of genetic testing for psychiatric disorders;
(c) investigation of family dynamics, the efficacy of psychological or other kind of educational and counseling intervention;
(d) hypothetical situations in undergoing genetic testing;
(e) direct to consumer genetic testing.
Recent reviews, meta-analyses or narrative accounts of knowledge were excluded.
For each study, we identified the implemented design, the number, and composition of participants, the psychological instruments employed and the main findings regarding psycho-behavioral outcomes and quality of life after testing.
Because of substantial heterogeneity among research studies, no attempt at formal meta-analysis was made in this contribution.
Studies Selection and Characteristics
Three thousand and three hundred twenty-eight manuscripts, published between 2000 and 2016, were assessed for eligibility by reading title and abstract. In total 90 studies were potentially eligible. After reading the full text, articles were included for qualitative synthesis only if they met inclusion/exclusion criteria. As a consequence, 43 studies could not be included, mostly because they investigated prenatal screening or childhood diseases, psychiatric disorders, family dynamics, the efficacy of the psychological intervention, genetic counseling effects or hypothetical situations in undergoing genetic testing. We considered these studies suitable to be treated as a separate topic.
Finally, a set of 47 studies met our inclusion criteria and were completely assessed (see Figure 1 for study selection and Table 1 for a summary of selected studies). Nine studies are concerned with cardiovascular diseases, 18 neurodegenerative disorders, and 20 cancer diseases. They had been conducted in the United States and Canada ( n = 22), Europe ( n = 22), Australia ( n = 1), Israel ( n = 1) and the islands of Flores and S.Miguel ( n = 1). Included studies have been classified according to the disease for which patients were tested (Table 1 first column), while the study design is reported in Table 2 .
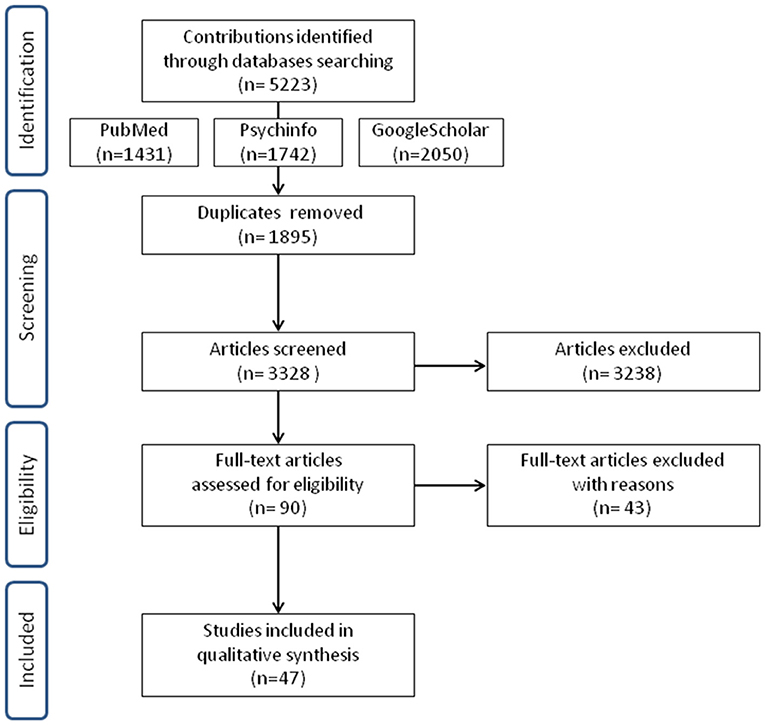
Figure 1 . Flow diagram describing the study selection.
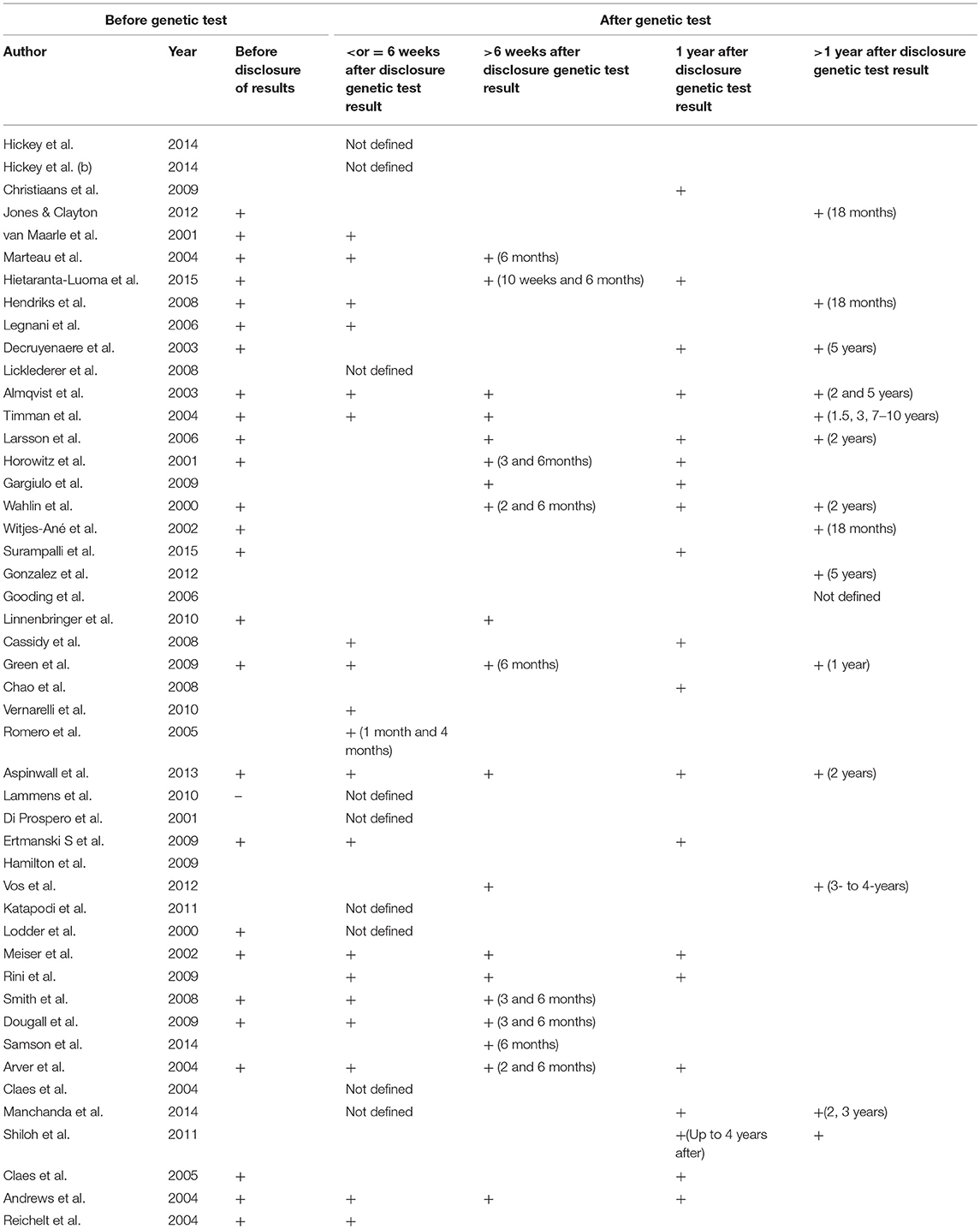
Table 2 . Moments of evaluation, before and after the genetic test, per study.
Cardiovascular Disease
Genetic testing for cardiovascular diseases is usually performed to detect users' susceptibility to conditions that affect the heart muscle, inherited heart conditions that might cause arrhythmias or risk factors which could cause a heart attack. Some of these conditions may require changes in lifestyle or medical therapy. Studies taken into examination evaluated the impact of genetic testing related to different clinical conditions ( Hickey et al., 2014a , b ), such as Long qt syndrome ( Hendriks et al., 2008 ), thrombophilia ( Legnani et al., 2006 ), cardiomyopathy ( Christiaans et al., 2009 ), arterial hypertension ( Jones and Clayton, 2012 ), and familial hypercholesterolaemia ( Van Maarle et al., 2001 ; Marteau et al., 2004 ). In general, these studies used similar scales for the assessment of the quality of life and perception of risk (SF-36, IPQ-R) and for evaluating the psychological impact and wellbeing (STAI, HADS, IES). Psychological aspects mainly concerned the anxiety-depressive symptoms and the subjective distress caused by the “traumatic event” of genetic risk communication.
Results showed that no negative impacts on quality of life and no serious increase in distress or anxiety levels were registered after receiving genetic test results ( Van Maarle et al., 2001 ; Marteau et al., 2004 ; Legnani et al., 2006 ; Hickey et al., 2014a , b ). Anxiety levels were overall moderate and tended to last over time only if the genetic test result was associated with uncertain physiological data ( Hendriks et al., 2008 ) or in case of marked clinical conditions, such as in patients with symptoms of hypertrophic cardiomyopathy before DNA testing ( Christiaans et al., 2009 ). Hietaranta-Luoma et al. (2015) evaluated the impact of genetic testing for the Apolipoprotein E (ApoE, a protein involved in Alzheimer's disease and cardiovascular disease and mapped to chromosome 19). They reported that in high-risk subjects the genetic information combined with personal health status influenced the levels of anxiety, and promoted the short-term reduction of risk factors for cardiovascular disease. One study investigated arterial hypertension ( Jones and Clayton, 2012 ) and detected distress symptoms in patients before undergoing genetic testing, comparable to PTSD symptoms. These symptoms significantly decreased after the reception of genetic results, in both carriers and non-carriers.
Even the quality of life after genetic test results was influenced by patients' difficulties in managing mental states, compared to other aspects. Hickey et al. (2014b) found that mental difficulties, assessed by the SF-36, were higher (59.9 ± 5.3) if compared to physical components, which resulted within normal ranges (46.2 ± 6.6), whereas Christiaans et al. (2009) clarified that levels of distress and impact on mental components did not significantly differ from the average of the general population who never underwent a genetic test. Finally, Marteau et al. (2004) showed that genetic testing for patients, already aware of their risk, does not affect their sense of control over the condition (hypercholesterolemia) but influenced their beliefs on how effectively achieve control on their health (e.g., with drug assumption).
Neurodegenerative Disorders
Genetic testing for neurodegenerative disorders are usually performed: (a) for diagnostic purposes, (b) to determine if a person, who has a family history of disease, is a mutation carrier and thus he/she is at risk to develop the disorder or could have an affected offspring. Currently, no therapies exist for complete remission of these pathologies. Studies we have collected primarily investigated risk related to Alzheimer disease (AD) and Huntington disease (HD). Two studies devoted attention to other neurodegenerative disorders, such as the Machado-Joseph disease (MJD)( Gonzalez et al., 2012 ) (which causes symptoms like spasticity, difficulty with speech and swallowing, weakness in arms and legs, frequent urination) and mutation to VCP gene ( Surampalli et al., 2015 ) (which along with the inclusion body myopathy it causes frontotemporal dementia).
Huntington Disease (HD) is a dominantly transmitted neurodegenerative disorder: genetic analysis detects, with 100% of certainty, the presence of the mutation gene, confirming the status as a carrier of the condition ( Evers-Kiebooms and Decruyenaere, 1998 ). The outcomes of gene testing can rarely fall within the reduced penetrance range (36–39 CAG repeats), whereby individuals may or may not develop symptoms of the disease; or individuals may be carriers of intermediate alleles (27–35 CAG repeats) and will not develop symptoms of the disease themselves, but their children will be at-risk of HD ( Myers, 2004 ).
Tools used to evaluate the psychological impact of genetic testing for HD predominantly measured anxiety and depression (STAI, BDI), the traumatic impact of the event “genetic test results communication” (IES) or severe psychological symptoms, up to suicidal ideations (see Table 1 ).
Most authors highlighted the presence of depression and suicidal ideation in a significant percentage of participants, even before undergoing genetic testing ( Robins Wahlin et al., 2000 ; Horowitz et al., 2001 ; Larsson et al., 2006 ; Gargiulo et al., 2009 ), with higher psychological suffering and negative impact on QoL for those with neurological symptoms ( Horowitz et al., 2001 ). Licklederer et al. (2008) found that patients, with mutation and already manifesting HD symptoms, had higher levels of depression and lower levels in QoL indexes, compared to gene carriers without symptoms and non-carriers. Moreover, they showed that depression values in HD gene carriers were related to unfavorable genetic test result in conjunction with negative social and relational conditions (e.g., low perceived social support and being childless). Summarizing, higher levels of depression and lower quality of life were registered in patients with manifest HD or neurological impairments ( Horowitz et al., 2001 ; Licklederer et al., 2008 ).
Interestingly, Gargiulo et al. (2009) found that 27% of non-carriers (asymptomatic) do not positively elaborate the favorable genetic results whereas Robins Wahlin et al. (2000) showed that non-carriers had a very high frequency of suicide ideations. Another study has also shown that non-carriers tended to develop avoidant or intrusive styles as a reaction to the stressful event (genetic test results) over time ( Timman et al., 2004 ).
Considering the long-term impact of genetic tests for HD, several studies revealed the presence or the increase in depressive symptoms, suicidal ideations, hopelessness, and aggressive reactions in gene carriers ( Robins Wahlin et al., 2000 ; Witjes-Ané et al., 2002 ; Almqvist et al., 2003 ; Timman et al., 2004 ; Larsson et al., 2006 ; Gargiulo et al., 2009 ), except for the study of Decruyenaere et al. (2003) , showing a significant decrease of depressive symptoms after 1 year, both in gene carriers and non-carriers.
Concerning Alzheimer disease (AD), currently ApoE testing is used in clinical settings to identify people who may have an increased risk of developing AD, whereas other genetic tests investigate the presence of autosomal dominant mutations (in genes PSEN1, PSEN2, and APP which are more predictive for disease development ( Goldman et al., 2011 ).
The REVEAL studies ( Chao et al., 2008 ; Green et al., 2009 ; Vernarelli et al., 2010 ) showed that ApoE carriers were not more anxious, depressed, or test-related distressed than people who did not receive any information about their genotype ( Green et al., 2009 ). The levels of anxiety, depression, and distress were below clinical thresholds both in carriers and non-carriers, with a significant distress reduction among those who learned that they were ApoE negative. People who were highly distressed before undergoing genetic testing were more vulnerable to emotional difficulties after outcome disclosure, but distress values were well below clinical thresholds for clinical concern ( Green et al., 2009 ). Romero et al. (2005) described that a small percentage of ApoE gene carriers felt depressed (15–30%) or worried (11–22%). A small percentage also felt relieved.
The study by Cassidy et al. (2008) found that participants who received a positive result for a deterministic mutation experienced the same levels of distress experienced by those receiving positive results for genetic susceptibility testing (ApoE). The same study reported that after 1 year from result disclosure the majority of participants did not experience clinically significant distress.
Concerning more in detail long-term results or changes in health-related behaviors, 12 months after ApoE results, carriers reported changes in lifestyle (diet, physical exercise, and medication or vitamin intake) more often than non-carriers or the nondisclosure group ( Chao et al., 2008 ). A positive correlation between genetic susceptibility testing for AD (an APOE epsilon4+ genotype status) and changes in vitamin intake was also confirmed by Vernarelli et al. (2010) , despite there is no evidence that supplement use reduces the risk of AD.
Finally, Linnenbringer et al. (2010) showed that people who accurately recalled their AD disease risk assessment (the risk percentage) tended to perceive their risk higher than the percentage of risk they were given (below clinical thresholds).
Finally, Gooding et al. (2006) interviewed a group of people at high risk for AD (because of relatives affected by AD), and genetic testing was estimated valuable information to improve personal control on health and guide future decisions.
In rare pathologies such as Machado Joseph disease ( Gonzalez et al., 2012 ), anxiety levels were from moderate to severe in half of the participants (52.6%). Five years later quality of life was significantly more compromised in symptomatic people, confirming an impact of the appearance of first symptoms on the psychological state. Meanwhile in VCP genetic testing ( Surampalli et al., 2015 ) were found similar results in anxiety levels as for Alzheimer disease.
Genetic testing for cancer is usually performed in pre-symptomatic conditions (the user never developed any symptom related to the cancer disease), or after an episode of cancer diagnosis, to know if there is a hereditary cancer syndrome and/or a risk of relapse.
Most of the articles focused on the risk of developing ovarian and breast cancer, by examining the presence of BRCA1 and BRCA2 mutations (see Table 1 ). Three studies, respectively, investigated the impact of genetic mutations responsible for pancreatic cancer and melanoma ( Aspinwall et al., 2013 ), colon cancer along with breast cancer ( Arver et al., 2004 ), and Li-Fraumeni Syndrome ( Lammens et al., 2010 ). Studies on BRCA testing used several tools (STAI, IES, SCL-90, BDI, HADS the most used ones) assessing anxiety, post-traumatic stress disorders, psychopathological symptoms and depression, and showed quite heterogeneous results.
Breast and ovarian cancer were overall perceived as having the same seriousness independently by genetic test results ( Claes et al., 2004 ). Many authors revealed the poor influence of genetic tests on anxiety and distress (distress levels within normal ranges), without significant difference between gene carriers and non-carriers ( Andrews et al., 2004 ; Claes et al., 2005 ; Ertmanski et al., 2009 ). These results suggest that genetic testing for BRCA does not cause adverse psychological reactions. Four studies reported slightly greater levels of anxiety and negative psychological outcomes in gene carriers ( Lodder et al., 2001a ; Meiser et al., 2002 ; Katapodi et al., 2011 ; Shiloh et al., 2013 ) whereas Vos et al. (2012) specified that these anxiety levels would be mediated by individual risk perception and concerns about their own relatives' heredity-likelihood. Gene carriers and probands showed to be more distressed and negatively influenced by genetic test results, even because they were concerned about their offspring and experienced decisional conflicts toward their relatives ( Claes et al., 2004 ; Rini et al., 2009 ; Katapodi et al., 2011 ). Three studies ( Reichelt et al., 2004 ; Ertmanski et al., 2009 ; Manchanda et al., 2015 ) investigated the experience of genetic testing and risk perception in people with a family history or personal history of illness, comparing them with healthy people or people without previous family experience of disease, and they found conflicting results. Manchanda et al. (2015) demonstrated that there were no differences in levels of anxiety and distress based on the presence/absence of a family history of disease, while Reichelt et al. (2004) and Ertmanski et al. (2009) reported higher levels of distress in people who have already had a diagnosis and/or cancer experience. Women with a personal cancer history tended to enact concrete coping strategies more than women without previous experience with cancer ( Dougall et al., 2009 ).
Finally, there are studies which reported satisfaction and positive consequences of having carried out the genetic test for breast/ovarian cancer susceptibility and thus discovering something about the presence of a mutation. In particular, receiving a positive result increased the perception of risk ( Di Prospero et al., 2001 ; Claes et al., 2005 ; Katapodi et al., 2011 ; Vos et al., 2012 ), which correlated with more frequent screenings and checkups, and with a sense of self-efficacy ( Di Prospero et al., 2001 ; Hamilton et al., 2009 ; Shiloh et al., 2013 ).
Long-term results showed that levels of test-related distress decreased in the first 4/6 months ( Andrews et al., 2004 ; Arver et al., 2004 ; Smith et al., 2008 ), then enduring at low levels after years ( Andrews et al., 2004 ; Manchanda et al., 2015 ), with an impact on surveillance actions up to 4 years after test disclosure ( Shiloh et al., 2013 ).
Concerning other cancers, in Aspinwall et al. (2013) gene carriers for pancreatic cancer and melanoma increased preventive screening for themselves and their families, thanks to informative genetic test results. A small percentage of patients reported clinically relevant levels of distress related to genetic testing for p53 germline mutation ( Lammens et al., 2010 ). Distress was higher for patients with a lack of social support, as was the case for Huntington Disease ( Licklederer et al., 2008 ).
In the last decades, clinical application of genetic testing for diagnosis and prevention has gained more importance to such an extent as to create a market where patients can obtain information on genetic risk in complete autonomy ( Su, 2013 ; Oliveri et al., 2015 , 2016b ; Oliveri and Pravettoni, 2016 ). In this framework, there are many possible psychological reactions and related issues worthy of consideration, such as risk perception and perceived controllability after a positive result for a mutation, or concerns about transmitting susceptibility for a disease to future generations.
With this contribution, we aimed to sound out possible differences in psychological reactions to predictive genetic testing based on different disease categories. To date, there are no reports that compare the psychological impact of genetic testing for cardiovascular, neurodegenerative and cancer diseases.
Our review shows that there is no significant increase in distress levels or adverse impact on the quality of life in subjects who undergo a genetic test for cardiovascular diseases; when higher distress is present it does not exceed the clinically significant threshold ( Van Maarle et al., 2001 ; Marteau et al., 2004 ; Legnani et al., 2006 ; Hickey et al., 2014b ). The psychological distress is related to a full-blown clinical condition in addition to a positive genetic result ( Hendriks et al., 2008 ; Christiaans et al., 2009 ). Overall people maintain confidence in being able to cope with their risk, even though they modify the opinion on how to address this risk: they tend to believe that lifestyle might be useless to face their “genetic predisposition,” and they need other “more concrete” methods of prevention, such as drug therapies ( Marteau et al., 2004 ). In our opinion, these trends arise from a “deterministic” interpretation of genetic data, and the lack of evidence concerning the effects of lifestyle modifications in the disease course. Changes in lifestyle only concern people who already have physical symptoms ( Marteau et al., 2004 ; Hietaranta-Luoma et al., 2015 ) and are at higher risk of adverse heart conditions, although these lifestyle changes have short duration ( Hietaranta-Luoma et al., 2015 ). We hypothesize that people with full-blown symptoms are motivated to gather all possible health-related information, including genetic risk information, in order to manage their risk of developing the disease. In general, our review shows that genetic risk for cardiovascular disease is perceived to be manageable, and this might also be due to the existence of screenings to prevent it and possible treatments.
Concerning neurodegenerative disorders, studies put more attention on anxiety and depression symptoms, since these disorders usually have relevance on complex emotions such as embarrassment and social withdrawal ( Levenson et al., 2014 ), affect family relations and put carriers at risk of social discrimination ( Perry, 1981 ; Craufurd and Harris, 1986 ). Our review describes marked negative psychological impact after positive genetic results for Huntington Disease in patients who have depressive symptoms already before undergoing genetic testing, including suicidal ideation, which are increased also by the presence of adverse relational/family situations ( Robins Wahlin et al., 2000 ; Horowitz et al., 2001 ; Larsson et al., 2006 ; Licklederer et al., 2008 ; Gargiulo et al., 2009 ). Differently from other chronic diseases, a negative genetic result for HD does not reassure, but it causes negative emotions. This reaction might be due to the uncertainty of results and a lack of “response” for the etiology of cognitive symptoms, when present, or sense of guilty toward family members that have the diseases ( Robins Wahlin et al., 2000 ; Timman et al., 2004 ; Gargiulo et al., 2009 ). However, we believe that these results for Huntington disease should be taken with the due caution called by the fact that, often, the evidence was based on participants with previous psychiatric history ( Robins Wahlin et al., 2000 ; Almqvist et al., 2003 ; Larsson et al., 2006 ; Gargiulo et al., 2009 ). Thus, we cannot rule out that, for example, a manifestation of suicidal ideation can be ascribable to this previous psychiatric history rather than to the positive or negative genetic result. Future studies should settle this issue.
Negative psychological impact of genetic testing in gene carriers for HD persists over time ( Almqvist et al., 2003 ; Timman et al., 2004 ; Larsson et al., 2006 ), and it might be due to the regret for having undergone the test, anticipating life change limitations ( Hagberg et al., 2011 ).
We argue that in addition to the regret for getting such genetic information, negative reactions may be understandable in light of the certainty these people have to develop HD in the future, the perception of something uncontrollable and fatal, alongside the complete absence of valid therapies and inevitable cognitive decline ( Gooding et al., 2006 ). The decision in undergoing predictive genetic testing, in this case, could be a coping strategy ( Gooding et al., 2006 )acted to redirect important life decisions.
Slightly different seems to be the impact of genetic analysis for Alzheimer disease. Effects of genetic test results are comparable to those described for cardiovascular diseases, since distress anxiety and depression are below clinically significant thresholds, even for gene carriers, and these results concern both APOE and autosomal dominant mutation testing ( Cassidy et al., 2008 ; Green et al., 2009 ; Linnenbringer et al., 2010 ). Therefore, the test is overall experienced as something useful to achieve a good degree of awareness and immediately act preventive behaviors to address the risk. Anyway, for Alzheimer's prevention behavioral changes are not always positive: for example, an increasing assumption of dietary supplements harmless, such as vitamin E, could give people a false perception of control on the health without any significant scientific evidence ( Morris et al., 2002 ). For this reason, it would be beneficial to provide people with more information on how to effectively prevent AD, before providing the opportunity to undergo genetic testing.
Finally, in rare diseases, such as Machado Joseph, anxiety levels were prominent after genetic testing in at least half of patients studied ( Gonzalez et al., 2012 ). These results are understandable in the light of an immediate impairment of daily life (e.g., spasticity, difficulty with speech and swallowing, weakness in arms and legs, frequent urination) and the fact that symptoms get worse over time.
Results on the impact of gene testing in cancer raise more complex and heterogeneous issues than in previous cases. From the emotional point of view, the levels of anxiety and depression decrease significantly after having received test results ( Andrews et al., 2004 ; Arver et al., 2004 ; Reichelt et al., 2004 ; Claes et al., 2005 ; Smith et al., 2008 ; Ertmanski et al., 2009 ; Lammens et al., 2010 ; Aspinwall et al., 2013 ; Manchanda et al., 2015 ), and a positive effect emerges as regards screening behaviors as well ( Hamilton et al., 2009 ; Shiloh et al., 2013 ). We must consider, in order to give an interpretation to the previous results, that breast and ovarian cancer are potentially preventable and early detection can guarantee to heal with effective treatments ( Shaw and Bassi, 2001 ). If results are positive, screening or surgery could help patients reduce their risks, and immediate communication to family members about genetic risk can be crucial to prevent the “danger” of disease development. Deciding on how to address the risk means being able to “recommend” a pathway for prevention to their families ( Lodder et al., 2001b ; Katapodi et al., 2011 ; Vos et al., 2012 ). Preventive and prophylactic decisional pathways are no easy nor straight: risk-reducing prophylactic mastectomy on healthy breast goes along the risk of surgical side effects, body image modification, regrets in women who decided for this solution. Periodic screening is instead potentially accompanied by frequent negative thoughts and emotions (anxiety components).
People who have already had an experience of illness tend to actively cope with the risk of disease onset, although sometimes this is accompanied by higher levels of distress ( Dougall et al., 2009 ). These levels of arousal, however, should not be necessarily perceived in a negative sense; on the contrary emotional arousal could be the engine for the decisional process in cancer care and for acting on coping strategies. Even for cancer, as already found for APOE and Alzheimer's, studies indicate that there are positive aspects reported by patients about having undergone genetic testing. These findings are related to an increase in screening behaviors and an increased sense of self-efficacy in managing the risk ( Hamilton et al., 2009 ; Aspinwall et al., 2013 ; Shiloh et al., 2013 ). Summarizing, in cancer, if people get important information on time they can manage their risky or healthy behaviors enhancing the perception of control over their lives and direct it as they wish (e.g., surgery vs . screening).
This is not completely true for cardiovascular disease and Huntington disease, because cardiovascular disease genetic information and evidence about preventive medicine efficacy are completely uncertain and say “something less” about predisposition and prevention options; for HD, deterministic implications of genetic testing give a piece of information that is likely to be “too much information,” and thus perceived as uncontrollable.
Before to conclude, it is important to point out some limitations of the reviewed studies. The first limitation is that gender was not well balanced in all the selected studies and was not investigated as a factor that could influence the psycho-behavioral impact of genetic testing. We exclude papers on breast cancer (which investigated women samples), Li-Fraumeni Syndrome (LFS) (where men and female were equally balanced and female gender was associated with heightened levels of LFS-related distress), and on the risk for developing diseases which disproportionately affect women (e.g., the primary pulmonary arterial hypertension)( Jones and Clayton, 2012 ). In many studies about neurodegenerative diseases, women were overrepresented ( Cassidy et al., 2008 ; Green et al., 2009 ; Vernarelli et al., 2010 ; Gonzalez et al., 2012 ) and we cannot exclude that the results could be affected by general gender bias. Chao et al. (2008) , for example, claimed that the REVEAL study participants were mainly women. Therefore the results may not be generalized to all population who might qualify for APOE genotype testing in the future.
In most studies concerning cardiovascular disease, males and female samples seemed to be well balanced, except for Hietaranta-Luoma et al. (2015) who evaluated more females. Nevertheless, gender differences, when investigated ( Legnani et al., 2006 ; Jones and Clayton, 2012 ; Hickey et al., 2014a ) did not relate to any of the measures of patient well-being.
A second limit was that not all the studies considered participants' educational level as a factor that could correlate with the decision to undergo a genetic test and its psycho-behavioral impact. In the selected studies participants had at least high school education (10–12 years of education completed)( Lodder et al., 2001b ; Van Maarle et al., 2001 ; Witjes-Ané et al., 2002 ; Almqvist et al., 2003 ; Claes et al., 2004 ; Gooding et al., 2006 ; Legnani et al., 2006 ; Licklederer et al., 2008 ; Smith et al., 2008 ; Christiaans et al., 2009 ; Rini et al., 2009 ; Vernarelli et al., 2010 ; Gonzalez et al., 2012 ; Vos et al., 2012 ; Hickey et al., 2014b ) or were predominantly highly educated ( Andrews et al., 2004 ; Claes et al., 2005 ; Dougall et al., 2009 ; Vernarelli et al., 2010 ; Aspinwall et al., 2013 ; Shiloh et al., 2013 ; Manchanda et al., 2015 ). Educational level was associated to a better recall of disease risk information ( Linnenbringer et al., 2010 ), to a higher response rates in the follow up ( Almqvist et al., 2003 ), or was not an influential predictor of the psycho-behavioral measures ( Robins Wahlin et al., 2000 ; Meiser et al., 2002 ; Andrews et al., 2004 ; Claes et al., 2005 ; Legnani et al., 2006 ; Licklederer et al., 2008 ; Smith et al., 2008 ; Green et al., 2009 ; Rini et al., 2009 ; Jones and Clayton, 2012 ; Shiloh et al., 2013 ). Other studies included in our review did not perform analysis based on the educational level, and future studies should address this issue.
Finally, some of the results presented in these studies are based on small sample sizes ( Robins Wahlin et al., 2000 ; Di Prospero et al., 2001 ; Andrews et al., 2004 ; Hamilton et al., 2009 ; Gonzalez et al., 2012 ; Hickey et al., 2014b ; Samson et al., 2014 ; Surampalli et al., 2015 ) and are not cross-cultural. Therefore, it is possible that ethnicity and cultural aspects may play a role in determining the psychological implications of genetic testing.
Conclusions
This review presented a comprehensive overview of the psychological impact of genetic testing across the most common chronic adults' diseases. The information level of genetic data varies according to the type of test. Along with this aspect, each of us has a specific perception of disease categories, for which genetic testing is available. Risk perception, worry, and other psychological reactions depend, for instance, on the perceived controllability and existing therapies to manage the illness; it is essential to proceed with an assessment of such factors along with the provision of genetic information.
Over the last 20 years we have witnessed a proliferation of investments in genomics research in order to study disease prevention, disease treatments, better drug therapies, and genetic paths to cure, and, thanks to media coverage such as Angelina Jolie's case ( Evans et al., 2014 ), the psychological impact of these discoveries has gradually become more and more important.
For these reasons, in the present review, we tried to understand better how genetic testing users' perceptions of developing specific diseases affect their psychological well-being and lifestyle. Understand psycho-behavioral reactions could be an important starting point for an effective clinical application of genetic testing and to organize personalized care plans, which can drive patients to self-determination of a healthy lifestyle and to make appropriate decisions for their health.
Author Contributions
SO and FF contributed to the design and implementation of the research, to the analysis of the results and the writing of the manuscript. AM contributed to the writing of the manuscript, and GP supervised all the process, was in charge of overall direction and planning.
Conflict of Interest Statement
The authors declare that the research was conducted in the absence of any commercial or financial relationships that could be construed as a potential conflict of interest.
Acknowledgments
This work is supported by a grant to the project Mind the Risk from The Swedish Foundation for Humanities and Social Sciences which did not influence the content of this paper. Grant nr. M13-0260:1.
Abegunde, D. O., Mathers, C. D., Adam, T., Ortegon, M., and Strong, K. (2007). The burden and costs of chronic diseases in low-income and middle-income countries. Lancet 370, 1929–1938. doi: 10.1016/S0140-6736(07)61696-1
PubMed Abstract | CrossRef Full Text | Google Scholar
Almqvist, E. W., Brinkman, R. R., Wiggins, S., and Hayden, M. R. (2003). Psychological consequences and predictors of adverse events in the first 5 years after predictive testing for Huntington's disease. Clin. Genet. 64, 300–309. doi: 10.1034/j.1399-0004.2003.00157.x
Andrews, L., Meiser, B., Apicella, C., and Tucker, K. (2004). Psychological impact of genetic testing for breast cancer susceptibility in women of Ashkenazi Jewish background: a prospective study. Genet. Test. 8, 240–247. doi: 10.1089/gte.2004.8.240
Arver, B., Haegermark, A., Platten, U., Lindblom, A., and Brandberg, Y. (2004). Evaluation of psychosocial effects of pre-symptomatic testing for breast/ovarian and colon cancer pre-disposing genes: a 12-month follow-up. Fam. Cancer 3, 109–116. doi: 10.1023/B:FAME.0000039863.89137.f9
Aspinwall, L. G., Taber, J. M., Leaf, S. L., Kohlmann, W., and Leachman, S. A. (2013). Genetic testing for hereditary melanoma and pancreatic cancer: a longitudinal study of psychological outcome. Psychooncology. 22, 276–289. doi: 10.1002/pon.2080
Burke, W., Atkins, D., Gwinn, M., Guttmacher, A., Haddow, J., Lau, J., et al. (2002). Genetic test evaluation: Information needs of clinicians, policy makers, and the public. Am. J. Epidemiol. 156, 311–318. doi: 10.1093/aje/kwf055
Cameron, L. D., and Muller, C. (2009). Psychosocial aspects of genetic testing. Curr. Opin. Psychiatry 22, 218–223. doi: 10.1097/YCO.0b013e3283252d80
Cassidy, M. R., Roberts, J. S., Bird, T. D., Steinbart, E. J., Cupples, L. A., Chen, C. A., et al. (2008). Comparing test-specific distress of susceptibility versus deterministic genetic testing for Alzheimer's disease. Alzheimer's Dement. 4, 406–413. doi: 10.1016/j.jalz.2008.04.007
Chao, S., Roberts, J. S., Marteau, T. M., Silliman, R., Cupples, L. A., and Green, R. C. (2008). Health behavior changes after genetic risk assessment for Alzheimer disease: The REVEAL Study. Alzheimer Dis. Assoc. Disord. 22, 94–97. doi: 10.1097/WAD.0b013e31815a9dcc
Christiaans, I., Van Langen, I. M., Birnie, E., Bonsel, G. J., Wilde, A. A. M., and Smets, E. M. A. (2009). Quality of life and psychological distress in hypertrophic cardiomyopathy mutation carriers: a cross-sectional cohort study. Am. J. Med. Genet. Part A 149, 602–612. doi: 10.1002/ajmg.a.32710
CrossRef Full Text | Google Scholar
Claes, E., Evers-Kiebooms, G., Boogaerts, A., Decruyenaere, M., Denayer, L., and Legius, E. (2004). Diagnostic genetic testing for hereditary breast and ovarian cancer in cancer patients: women's looking back on the pre-test period and a psychological evaluation. Genet. Test. 8, 13–21. doi: 10.1089/109065704323015996
Claes, E., Evers-Kiebooms, G., Denayer, L., Decruyenaere, M., Boogaerts, A., Philippe, K., et al. (2005). Predictive genetic testing for hereditary breast and ovarian cancer: psychological distress and illness representations 1 year following disclosure. J. Genet. Couns. 14, 349–363. doi: 10.1007/s10897-005-1371-4
Collins, F. S., and McKusick, V. A. (2001). Implications of the Human Genome Project for medical science. JAMA 285, 540–544. doi: 10.1001/jama.285.5.540
Cornel, M. C., Van El, C. G., and Borry, P. (2014). The challenge of implementing genetic tests with clinical utility while avoiding unsound applications. J. Community Genet. 5, 7–12. doi: 10.1007/s12687-012-0121-1
Craufurd, D. I., and Harris, R. (1986). Ethics of predictive testing for Huntington's chorea: the need for more information. Br. Med. J. (Clin. Res. Ed). 293, 249–251.
PubMed Abstract | Google Scholar
Curtis, C., Shah, S. P., Chin, S.-F., Turashvili, G., Rueda, O. M., Dunning, M. J., et al. (2012). The genomic and transcriptomic architecture of 2,000 breast tumours reveals novel subgroups. Nature 486, 346–352. doi: 10.1038/nature10983
Decruyenaere, M., Evers-Kiebooms, G., Cloostermans, T., Boogaerts, A., Demyttenaere, K., Dom, R., et al. (2003). Psychological distress in the 5-year period after predictive testing for Huntington's disease. Eur. J. Hum. Genet . 11, 30–38. doi: 10.1038/sj.ejhg.5200913
Désesquelles, A., Demuru, E., Pappagallo, M., Salvatore, M. A., Frova, L., Meslé, F., et al. (2014). Mortality from Alzheimer' s disease, Parkinson' s disease, and dementias in france and Italy : a comparison using the multiple approach. J. Aging Health 26, 283–315. doi: 10.1177/0898264313514443
Di Prospero, L. S., Seminsky, M., Honeyford, J., Doan, B., Franssen, E., Meschino, W., et al. (2001). Psychosocial issues following a positive result of genetic testing for BRCA1 and BRCA2 mutations: findings from a focus group and a needs-assessment survey. CMAJ 164, 1005–1009. doi: 10.1002/ijc.23340
DiLorenzo, T. A., Schnur, J., Montgomery, G. H., Erblich, J., Winkel, G., and Bovbjerg, D. H. (2006). A model of disease-specific worry in heritable disease: The influence of family history, perceived risk and worry about other illnesses. J. Behav. Med. 29, 37–49. doi: 10.1007/s10865-005-9039-y
Dougall, A. L., Smith, A. W., Somers, T. J., Posluszny, D. M., Rubinstein, W. S., and Baum, A. (2009). Coping with genetic testing for breast cancer susceptibility. Psychosom. Med. 71, 98–105. doi: 10.1097/PSY.0b013e318190d7b4
Erkkinen, M. G., Kim, M.-O., and Geschwind, M. D. (2018). Clinical neurology and epidemiology of the major neurodegenerative diseases. Cold Spring Harb. Perspect. Biol. 10:a033118. doi: 10.1101/cshperspect.a033118
Ertmanski, S.ł., Metcalfe, K., TrempaŁa, J., GŁowacka, M. D., Lubinski, J., Narod, S. A., et al. (2009). Identification of patients at high risk of psychological distress after BRCA1 genetic testing. Genet. Test. Mol. Biomarkers 13, 325–330. doi: 10.1089/gtmb.2008.0126
Evans, D. G., Barwell, J., Eccles, D. M., Collins, A., Izatt, L., Jacobs, C., et al. (2014). The Angelina Jolie effect: how high celebrity profile can have a major impact on provision of cancer related services. Breast Cancer Res. 16, 442. doi: 10.1186/s13058-014-0442-6
Evers-Kiebooms, G., and Decruyenaere, M. (1998). Predictive testing for Huntington's disease: a challenge for persons at risk and for professionals. Patient Educ. Couns. 35, 15–26.
Ferlay, J., Soerjomataram, I., Dikshit, R., Eser, S., Mathers, C., Rebelo, M., et al. (2015). Cancer incidence and mortality worldwide: Sources, methods and major patterns in GLOBOCAN 2012. Int. J. Cancer 136, E359–E386. doi: 10.1002/ijc.29210
Gargiulo, M., Lejeune, S., Tanguy, M.-L., Lahlou-Laforêt, K., Faudet, A., Cohen, D., et al. (2009). Long-term outcome of presymptomatic testing in Huntington disease. Eur. J. Hum. Genet. 17, 165–171. doi: 10.1038/ejhg.2008.146
Giardino, D., Mingarelli, R., Lauretti, T., Amoroso, A., Larizza, L., and Dallapiccola, B. (2016). Survey of medical genetic services in Italy: year 2011. BMC Health Serv. Res. 16:96. doi: 10.1186/s12913-016-1340-7
Goldman, J. S., Hahn, S. E., Catania, J. W., LaRusse-Eckert, S., Butson, M. B., Rumbaugh, M., et al. (2011). Genetic counseling and testing for Alzheimer disease: joint practice guidelines of the American College of Medical Genetics and the National Society of Genetic Counselors. Genet. Med. 13, 597–605. doi: 10.1097/GIM.0b013e31821d69b8
Gonzalez, C., Gomes, E., Kazachkova, N., Bettencourt, C., Raposo, M., Kay, T. T., et al. (2012). Psychological well-being and family satisfaction levels five years after being confirmed as a carrier of the Machado-Joseph disease mutation. Genet. Test. Mol. Biomarkers 16, 1363–1368. doi: 10.1089/gtmb.2011.0370
Gooding, H. C., Linnenbringer, E. L., Burack, J., Roberts, J. S., Green, R. C., and Biesecker, B. B. (2006). Genetic susceptibility testing for Alzheimer disease: Motivation to obtain information and control as precursors to coping with increased risk. Patient Educ. Couns. 64, 259–267. doi: 10.1016/j.pec.2006.03.002
Green, R. C., Roberts, J. S., Cupples, L. A., Relkin, N. R., Whitehouse, P. J., Brown, T., et al. (2009). Disclosure of APOE genotype for risk of Alzheimer's disease. N. Engl. J. Med. 361, 245–254. doi: 10.1056/NEJMoa0809578
Hagberg, A., Bui, T. H., and Winnberg, E. (2011). More appreciation of life or regretting the test? Experiences of living as a mutation carrier of Huntington's disease. J. Genet. Couns. 20, 70–79. doi: 10.1007/s10897-010-9329-6
Hamilton, R., Williams, J. K., Skirton, H., and Bowers, B. J. (2009). Living with genetic test results for hereditary breast and ovarian cancer. J. Nurs. Scholarsh. 41, 276–283. doi: 10.1111/j.1547-5069.2009.01279.x
Hendriks, K. S. W. H., Hendriks, M. M. W. B., Birnie, E., Grosfeld, F. J. M., Wilde, A., van den Bout, J., et al. (2008). Familial disease with a risk of sudden death: a longitudinal study of the psychological consequences of predictive testing for long QT syndrome. Heart Rhythm 5, 719–724. doi: 10.1016/j.hrthm.2008.01.032
Heshka, J. T., Palleschi, C., Howley, H., Wilson, B., and Wells, P. S. (2008). A systematic review of perceived risks, psychological and behavioral impacts of genetic testing. Genet. Med. 10, 19–32. doi: 10.1097/GIM.0b013e31815f524f
Hickey, K. T., Sciacca, R. R., Biviano, A. B., Whang, W., Dizon, J. M., Garan, H., et al. (2014a). The effect of cardiac genetic testing on psychological well-being and illness perceptions. Hear. Lung J. Acute Crit. Care 43, 127–132. doi: 10.1016/j.hrtlng.2014.01.006
Hickey, K. T., Taylor, J. Y., Sciacca, R. R., Aboelela, S., Gonzalez, P., Castillo, C., et al. (2014b). Cardiac genetic testing: a single-center pilot study of a Dominican population. Hisp. Health Care Int. 12, 183–188. doi: 10.1891/1540-4153.12.4.183
Hietaranta-Luoma, H.-L., Luomala, H. T., Puolijoki, H., and Hopia, A. (2015). Using ApoE genotyping to promote healthy lifestyles in Finland–psychological impacts: randomized controlled trial. J. Genet. Couns. 24, 908–921. doi: 10.1007/s10897-015-9826-8
Hollands, G. J., French, D. P., Griffin, S. J., Prevost, A. T., Sutton, S., King, S., et al. (2016). The impact of communicating genetic risks of disease on riskreducing health behaviour: Systematic review with meta-analysis. BMJ 352. doi: 10.1136/bmj.i1102
Horowitz, M. J., Field, N. P., Zanko, A., Donnelly, E. F., Epstein, C., and Longo, F. (2001). Psychological impact of news of genetic risk for Huntington disease. Am. J. Med. Genet. 103, 188–192. doi: 10.1002/ajmg.1538
Janssens, S., Chokoshvili, D., Vears, D. F., De Paepe, A., and Borry, P. (2017). Pre- and post-testing counseling considerations for the provision of expanded carrier screening: Exploration of European geneticists' views. BMC Med. Ethics 18:46. doi: 10.1186/s12910-017-0206-9
Jones, D. L., and Clayton, E. W. (2012). The role of distress in uptake and response to predisposition genetic testing: the BMPR2 experience. Genet. Test. Mol. Biomarkers 16, 203–209. doi: 10.1089/gtmb.2011.0059
Katapodi, M. C., Northouse, L., Pierce, P., Milliron, K. J., Liu, G., and Merajver, S. D. (2011). Differences between women who pursued genetic testing for hereditary breast and ovarian cancer and their at-risk relatives who did not. Oncol. Nurs. Forum 38, 572–581. doi: 10.1188/11.ONF.572-581
Khoury, M. J., Yang, Q., Gwinn, M., Little, J., and Flanders, W. D. (2004). An epidemiologic assessment of genomic profiling for measuring susceptibility to common diseases and targeting interventions. Genet. Med. 6, 38–47. doi: 10.1097/01.GIM.0000105751.71430.79
Lammens, C. R. M., Aaronson, N. K., Wagner, A., Sijmons, R. H., Ausems, M. G. E. M., Vriends, A. H. J. T., et al. (2010). Genetic testing in Li-Fraumeni syndrome: Uptake and psychosocial consequences. J. Clin. Oncol. 28, 3008–3014. doi: 10.1200/JCO.2009.27.2112
Larsson, M. U., Luszcz, M., a, Bui, T.-H., and Wahlin, T.-B. R. (2006). Depression and suicidal ideation after predictive testing for Huntington's disease: a two-year follow-up study. J. Genet. Couns. 15, 361–374. doi: 10.1007/s10897-006-9027-6
Legnani, C., Razzaboni, E., Gremigni, P., Bitti, P. E. R., Favaretto, E., and Palareti, G. (2006). Psychological impact of testing for thrombophilic alterations. Thromb. Haemost. 96, 348–355. doi: 10.1160/TH06-01-0015
Levenson, R. W., Sturm, V. E., and Haase, C. M. (2014). Emotional and behavioral symptoms in neurodegenerative disease: a model for studying the neural bases of psychopathology. Annu. Rev. Clin. Psychol. 10, 581–606. doi: 10.1146/annurev-clinpsy-032813-153653
Licklederer, C., Wolff, G., and Barth, J. (2008). Mental health and quality of life after genetic testing for huntington disease: a long-term effect study in Germany. Am. J. Med. Genet. Part A 146, 2078–2085. doi: 10.1002/ajmg.a.32423
Linnenbringer, E., Roberts, J. S., Hiraki, S., Cupples, L. A., and Green, R. C. (2010). “I know what you told me, but this is what I think:” perceived risk of Alzheimer disease among individuals who accurately recall their genetics-based risk estimate. Genet. Med. 12, 219–227. doi: 10.1097/GIM.0b013e3181cef9e1
Lodder, L., Frets, P. G., Trijsburg, R. W., Meijers-Heijboer, E. J., Klijn, J. G. M., Duivenvoorden, H. J., et al. (2001a). Psychological impact of receiving a BRCA1/BRCA2 test result. Am. J. Med. Genet. Part A 98, 15–24.
Lodder, L., Frets, P. G., Willem Trijsburg, R., Johanna Meijers-Heijboer, E., Klijn, J. G. M., Duivenvoorden, H. J., et al. (2001b). Psychological impact of receiving a BRCA1/BRCA2 test result. Am. J. Med. Genet. 98, 15–24. doi: 10.1002/1096-8628(20010101)98:1<15::AID-AJMG1014>3.0.CO;2-0
Mackenbach, J. P., Karanikolos, M., and Looman, C. W. N. (2014). The rise of mortality from mental and neurological diseases in Europe, 1979-2009: observational study. BMC Public Health 14:840. doi: 10.1186/1471-2458-14-840
Manchanda, R., Loggenberg, K., Sanderson, S., Burnell, M., Wardle, J., Gessler, S., et al. (2015). Population testing for cancer predisposing BRCA1/BRCA2 mutations in the ashkenazi-jewish community: a randomized controlled trial. J. Natl. Cancer Inst. 107. doi: 10.1093/jnci/dju379
Marteau, T., Senior, V., Humphries, S. E., Bobrow, M., Cranston, T., Crook, M., et al. (2004). Psychological impact of genetic testing for familial hypercholesterolemia within a previously aware population: a randomized controlled trial. Am. J. Med. Genet. A 128A, 285–293. doi: 10.1002/ajmg.a.30102
Meiser, B., Butow, P., Friedlander, M., Barratt, A., Schnieden, V., Watson, M., et al. (2002). Psychological impact of genetic testing in women from high-risk breast cancer families. Eur. J. Cancer 38, 2025–2031. doi: 10.1016/S0959-8049(02)00264-2
Morris, M. C., Evans, D., a, Bienias, J. L., Tangney, C. C., Bennett, D. a, Aggarwal, N., et al. (2002). Dietary intake of antioxidant nutrients and the risk of incident Alzheimer disease in a biracial community study. JAMA 287, 3230–3237. doi: 10.1001/jama.287.24.3230
Myers, R. H. (2004). Huntington' s Disease Genetics. Am. Soc. Exp. Neurother. 1, 255–262.
Google Scholar
Oliveri, S., Howard, H. C., Renzi, C., Hansson, M. G., and Pravettoni, G. (2016a). Anxiety delivered direct-to-consumer: are we asking the right questions about the impacts of DTC genetic testing? J. Med. Genet. 53, 798–799. doi: 10.1136/jmedgenet-2016-104184
Oliveri, S., and Pravettoni, G. (2016). The disclosure of direct-to-consumer genetic testing: sounding out the psychological perspective of consumers. Biol. Med. 8, 1–4. doi: 10.4172/0974-8369.1000316
Oliveri, S., Pravettoni, G., Fioretti, C., and Hansson, M. G. (2016b). Let the individuals directly concerned decide: a solution to tragic choices in genetic risk information. Public Health Genomics 19, 307–313. doi: 10.1159/000448913
Oliveri, S., Renzi, C., Masiero, M., and Pravettoni, G. (2015). Living at risk: factors that affect the experience of direct-to-consumer genetic testing. Mayo Clin. Proc. 90, 1323–1326. doi: 10.1016/j.mayocp.2015.06.014
Perry, T. L. (1981). Some ethical problems in Huntington's chorea. Can. Med. Assoc. J. 125, 1098.
Reichelt, J. G., Heimdal, K., Møller, P., and Dahl, A. A. (2004). BRCA1 testing with definitive results: a prospective study of psychological distress in a large clinic-based sample. Fam. Cancer 3, 21–28. doi: 10.1023/B:FAME.0000026820.32469.4a
Rini, C., O'Neill, S. C., Valdimarsdottir, H., Goldsmith, R. E., Jandorf, L., Brown, K., et al. (2009). Cognitive and emotional factors predicting decisional conflict among high-risk breast cancer survivors who receive uninformative BRCA1/2 results. Health Psychol. 28, 569–578. doi: 10.1037/a0015205
Robins Wahlin, T. B., Backman, L., Lundin, A., Haegermark, A., Winblad, B., and Anvret, M. (2000). High suicidal ideation in persons testing for Huntington's disease. Acta Neurol Scand 102, 150–161. doi: 10.1034/j.1600-0404.2000.102003150.x
Romero, L. J., Garry, P. J., Schuyler, M., Bennahum, D. A., Qualls, C., Ballinger, L., et al. (2005). Emotional responses to APO E genotype disclosure for Alzheimer disease. J. Genet. Couns . 14, 141–150. doi: 10.1007/s10897-005-4063-1
Roth, G. A., Johnson, C., Abajobir, A., Abd-Allah, F., Abera, S. F., Abyu, G., et al. (2017). Global, regional, and national burden of cardiovascular diseases for 10 causes, 1990 to 2015. J. Am. Coll. Cardiol. 70, 1–25. doi: 10.1016/j.jacc.2017.04.052
Samson, A., DiMillo, J., Thériault, A., Lowry, S., Corsini, L., Verma, S., et al. (2014). Living with the BRCA1 and BRCA2 genetic mutation: Learning how to adapt to a virtual chronic illness. Psychol. Health Med. 19, 103–114. doi: 10.1080/13548506.2013.779729
Shaw, J. S., and Bassi, K. L. (2001). Lay attitudes toward genetic Testing for susceptibility to inherited diseases. J. Health Psychol. 6, 405–423. doi: 10.1177/135910530100600404
Shiloh, S., Dagan, E., Friedman, I., Blank, N., and Friedman, E. (2013). A follow-up study on men tested for BRCA1/BRCA2 mutations: impacts and coping processes. Psychooncology. 22, 417–425. doi: 10.1002/pon.2106
Smith, A. W., Dougall, A. L., Posluszny, D. M., Somers, T. J., Rubinstein, W. S., and Baum, A. (2008). Psychological distress and quality of life associated with genetic testing for breast cancer risk. Psychooncology 17, 767–773. doi: 10.1002/pon.1291
Su, P. (2013). Direct-to-consumer genetic testing: a comprehensive view. Yale J. Biol. Med. 86, 359–365.
Surampalli, A., Khare, M., Kubrussi, G., Wencel, M., Tanaja, J., Donkervoort, S., et al. (2015). Psychological impact of predictive genetic testing in VCP inclusion body myopathy, paget disease of bone and frontotemporal dementia. J. Genet. Couns. 24, 842–850. doi: 10.1007/s10897-015-9819-7
Suzman, R., Beard, J. R., Boerma, T., and Chatterji, S. (2015). Health in an ageing world—what do we know? Lancet 385, 484–486. doi: 10.1016/S0140-6736(14)61597-X
Timman, R., Roos, R., Maat-Kievit, A., and Tibben, A. (2004). Adverse effects of predictive testing for Huntington disease underestimated: long-term effects 7-10 years after the test. Health Psychol. 23, 189–197. doi: 10.1037/0278-6133.23.2.189
Van Maarle, M. C., Stouthard, M. E. A., d. Mheen, P. J., Klazinga, N. S., and Bonsel, G. J. (2001). How disturbing is it to be approached for a genetic cascade screening programme for familial hypercholesterolaemia?: psychological impact and screenees' views. Community Genet. 4, 244–252. doi: 10.1159/000064200
Vernarelli, J. A., Roberts, J. S., Hiraki, S., Chen, C. A., Cupples, L. A., and Green, R. C. (2010). Effect of Alzheimer disease genetic risk disclosure on dietary supplement use. Am. J. Clin. Nutr. 91, 1402–1407. doi: 10.3945/ajcn.2009.28981
Vos, J., Oosterwijk, J. C., Gomez-Garcia, E., Menko, F. H., Collee, M. J., van Asperen, C. J., et al. (2012). Exploring the short-term impact of DNA-testing in breast cancer patients: The counselees' perception matters, but the actual BRCA1/2 result does not. Patient Educ. Couns. 86, 239–251. doi: 10.1016/j.pec.2011.04.017
Wade, C. H., Shiloh, S., Woolford, S. W., Roberts, J. S., Alford, S. H., Marteau, T. M., et al. (2012). Modelling decisions to undergo genetic testing for susceptibility to common health conditions: an ancillary study of the Multiplex Initiative. Psychol. Health 27, 430–444. doi: 10.1080/08870446.2011.586699
Walter, F. M., and Emery, J. D. (2012). Genetic advances in medicine: has the promise been fulfilled in general practice? Br. J. Gen. Pract . 62, 120–121. doi: 10.3399/bjgp12X629955
Wang, C., O'Neill, S. M., Rothrock, N., Gramling, R., Sen, A., Acheson, L. S., et al. (2009). Comparison of risk perceptions and beliefs across common chronic diseases. Prev. Med. (Baltim). 48, 197–202. doi: 10.1016/j.ypmed.2008.11.008
WHO and FAO (2003). Diet, Nutrition and the Prevention of Chronic Diseases . WHO and FAO.
Witjes-Ané, M. N. W., Zwinderman, A. H., Tibben, A., van Ommen, G. J. B., and Roos, R. A. C. (2002). Behavioural complaints in participants who underwent predictive testing for Huntington's disease. J. Med. Genet. 39, 857–862. doi: 10.1136/jmg.39.11.857
World Health Organisation (2014). Diet, Nutrition and the Prevention of Chronic Diseases. WHO Tech. Rep. Ser. No. 916 . Available online at: http://www.who.int/dietphysicalactivity/publications/trs916/summary/en/
Yang, Q., Khoury, M. J., Botto, L., Friedman, J. M., and Flanders, W. D. (2003). Improving the prediction of complex diseases by testing for multiple disease-susceptibility genes. Am. J. Hum. Genet. 72, 636–649. doi: 10.1086/367923
Yoon, P. (2005). CDC's family history public health initiative: 2005 update in Genomics and Population Health 2005 , eds M. Gwinn, S. Bedrosian, D. Ottman, and M. J. Khoury (Atlanta, GA: Centers for Disease Control and Prevention, Office of Genomics and Disease Prevention), 11–13.
Yoon, P., and Scheuner, M. (2003). The family history public health initiative in Genomics and Population Health: United States 2004 , eds M. Gwinn, S. Bedrosian, D. Ottman, and M. J. Khoury (Atlanta, GA: Centers for Disease Control and Prevention, Office of Genomics and Disease Prevention), 39–45.
Keywords: genetic testing, genetic risk, chronic disease, psychological implication, quality of life, health psychology
Citation: Oliveri S, Ferrari F, Manfrinati A and Pravettoni G (2018) A Systematic Review of the Psychological Implications of Genetic Testing: A Comparative Analysis Among Cardiovascular, Neurodegenerative and Cancer Diseases. Front. Genet . 9:624. doi: 10.3389/fgene.2018.00624
Received: 17 October 2017; Accepted: 23 November 2018; Published: 10 December 2018.
Reviewed by:
Copyright © 2018 Oliveri, Ferrari, Manfrinati and Pravettoni. This is an open-access article distributed under the terms of the Creative Commons Attribution License (CC BY) . The use, distribution or reproduction in other forums is permitted, provided the original author(s) and the copyright owner(s) are credited and that the original publication in this journal is cited, in accordance with accepted academic practice. No use, distribution or reproduction is permitted which does not comply with these terms.
*Correspondence: Serena Oliveri, [email protected]
Disclaimer: All claims expressed in this article are solely those of the authors and do not necessarily represent those of their affiliated organizations, or those of the publisher, the editors and the reviewers. Any product that may be evaluated in this article or claim that may be made by its manufacturer is not guaranteed or endorsed by the publisher.
- Skip to main content
- Keyboard shortcuts for audio player
NPR defends its journalism after senior editor says it has lost the public's trust

David Folkenflik

NPR is defending its journalism and integrity after a senior editor wrote an essay accusing it of losing the public's trust. Saul Loeb/AFP via Getty Images hide caption
NPR is defending its journalism and integrity after a senior editor wrote an essay accusing it of losing the public's trust.
NPR's top news executive defended its journalism and its commitment to reflecting a diverse array of views on Tuesday after a senior NPR editor wrote a broad critique of how the network has covered some of the most important stories of the age.
"An open-minded spirit no longer exists within NPR, and now, predictably, we don't have an audience that reflects America," writes Uri Berliner.
A strategic emphasis on diversity and inclusion on the basis of race, ethnicity and sexual orientation, promoted by NPR's former CEO, John Lansing, has fed "the absence of viewpoint diversity," Berliner writes.
NPR's chief news executive, Edith Chapin, wrote in a memo to staff Tuesday afternoon that she and the news leadership team strongly reject Berliner's assessment.
"We're proud to stand behind the exceptional work that our desks and shows do to cover a wide range of challenging stories," she wrote. "We believe that inclusion — among our staff, with our sourcing, and in our overall coverage — is critical to telling the nuanced stories of this country and our world."

NPR names tech executive Katherine Maher to lead in turbulent era
She added, "None of our work is above scrutiny or critique. We must have vigorous discussions in the newsroom about how we serve the public as a whole."
A spokesperson for NPR said Chapin, who also serves as the network's chief content officer, would have no further comment.
Praised by NPR's critics
Berliner is a senior editor on NPR's Business Desk. (Disclosure: I, too, am part of the Business Desk, and Berliner has edited many of my past stories. He did not see any version of this article or participate in its preparation before it was posted publicly.)
Berliner's essay , titled "I've Been at NPR for 25 years. Here's How We Lost America's Trust," was published by The Free Press, a website that has welcomed journalists who have concluded that mainstream news outlets have become reflexively liberal.
Berliner writes that as a Subaru-driving, Sarah Lawrence College graduate who "was raised by a lesbian peace activist mother ," he fits the mold of a loyal NPR fan.
Yet Berliner says NPR's news coverage has fallen short on some of the most controversial stories of recent years, from the question of whether former President Donald Trump colluded with Russia in the 2016 election, to the origins of the virus that causes COVID-19, to the significance and provenance of emails leaked from a laptop owned by Hunter Biden weeks before the 2020 election. In addition, he blasted NPR's coverage of the Israel-Hamas conflict.
On each of these stories, Berliner asserts, NPR has suffered from groupthink due to too little diversity of viewpoints in the newsroom.
The essay ricocheted Tuesday around conservative media , with some labeling Berliner a whistleblower . Others picked it up on social media, including Elon Musk, who has lambasted NPR for leaving his social media site, X. (Musk emailed another NPR reporter a link to Berliner's article with a gibe that the reporter was a "quisling" — a World War II reference to someone who collaborates with the enemy.)
When asked for further comment late Tuesday, Berliner declined, saying the essay spoke for itself.
The arguments he raises — and counters — have percolated across U.S. newsrooms in recent years. The #MeToo sexual harassment scandals of 2016 and 2017 forced newsrooms to listen to and heed more junior colleagues. The social justice movement prompted by the killing of George Floyd in 2020 inspired a reckoning in many places. Newsroom leaders often appeared to stand on shaky ground.
Leaders at many newsrooms, including top editors at The New York Times and the Los Angeles Times , lost their jobs. Legendary Washington Post Executive Editor Martin Baron wrote in his memoir that he feared his bonds with the staff were "frayed beyond repair," especially over the degree of self-expression his journalists expected to exert on social media, before he decided to step down in early 2021.
Since then, Baron and others — including leaders of some of these newsrooms — have suggested that the pendulum has swung too far.

Author Interviews
Legendary editor marty baron describes his 'collision of power' with trump and bezos.
New York Times publisher A.G. Sulzberger warned last year against journalists embracing a stance of what he calls "one-side-ism": "where journalists are demonstrating that they're on the side of the righteous."
"I really think that that can create blind spots and echo chambers," he said.
Internal arguments at The Times over the strength of its reporting on accusations that Hamas engaged in sexual assaults as part of a strategy for its Oct. 7 attack on Israel erupted publicly . The paper conducted an investigation to determine the source of a leak over a planned episode of the paper's podcast The Daily on the subject, which months later has not been released. The newsroom guild accused the paper of "targeted interrogation" of journalists of Middle Eastern descent.
Heated pushback in NPR's newsroom
Given Berliner's account of private conversations, several NPR journalists question whether they can now trust him with unguarded assessments about stories in real time. Others express frustration that he had not sought out comment in advance of publication. Berliner acknowledged to me that for this story, he did not seek NPR's approval to publish the piece, nor did he give the network advance notice.
Some of Berliner's NPR colleagues are responding heatedly. Fernando Alfonso, a senior supervising editor for digital news, wrote that he wholeheartedly rejected Berliner's critique of the coverage of the Israel-Hamas conflict, for which NPR's journalists, like their peers, periodically put themselves at risk.
Alfonso also took issue with Berliner's concern over the focus on diversity at NPR.
"As a person of color who has often worked in newsrooms with little to no people who look like me, the efforts NPR has made to diversify its workforce and its sources are unique and appropriate given the news industry's long-standing lack of diversity," Alfonso says. "These efforts should be celebrated and not denigrated as Uri has done."
After this story was first published, Berliner contested Alfonso's characterization, saying his criticism of NPR is about the lack of diversity of viewpoints, not its diversity itself.
"I never criticized NPR's priority of achieving a more diverse workforce in terms of race, ethnicity and sexual orientation. I have not 'denigrated' NPR's newsroom diversity goals," Berliner said. "That's wrong."
Questions of diversity
Under former CEO John Lansing, NPR made increasing diversity, both of its staff and its audience, its "North Star" mission. Berliner says in the essay that NPR failed to consider broader diversity of viewpoint, noting, "In D.C., where NPR is headquartered and many of us live, I found 87 registered Democrats working in editorial positions and zero Republicans."
Berliner cited audience estimates that suggested a concurrent falloff in listening by Republicans. (The number of people listening to NPR broadcasts and terrestrial radio broadly has declined since the start of the pandemic.)
Former NPR vice president for news and ombudsman Jeffrey Dvorkin tweeted , "I know Uri. He's not wrong."
Others questioned Berliner's logic. "This probably gets causality somewhat backward," tweeted Semafor Washington editor Jordan Weissmann . "I'd guess that a lot of NPR listeners who voted for [Mitt] Romney have changed how they identify politically."
Similarly, Nieman Lab founder Joshua Benton suggested the rise of Trump alienated many NPR-appreciating Republicans from the GOP.
In recent years, NPR has greatly enhanced the percentage of people of color in its workforce and its executive ranks. Four out of 10 staffers are people of color; nearly half of NPR's leadership team identifies as Black, Asian or Latino.
"The philosophy is: Do you want to serve all of America and make sure it sounds like all of America, or not?" Lansing, who stepped down last month, says in response to Berliner's piece. "I'd welcome the argument against that."
"On radio, we were really lagging in our representation of an audience that makes us look like what America looks like today," Lansing says. The U.S. looks and sounds a lot different than it did in 1971, when NPR's first show was broadcast, Lansing says.
A network spokesperson says new NPR CEO Katherine Maher supports Chapin and her response to Berliner's critique.
The spokesperson says that Maher "believes that it's a healthy thing for a public service newsroom to engage in rigorous consideration of the needs of our audiences, including where we serve our mission well and where we can serve it better."
Disclosure: This story was reported and written by NPR Media Correspondent David Folkenflik and edited by Deputy Business Editor Emily Kopp and Managing Editor Gerry Holmes. Under NPR's protocol for reporting on itself, no NPR corporate official or news executive reviewed this story before it was posted publicly.
- International edition
- Australia edition
- Europe edition
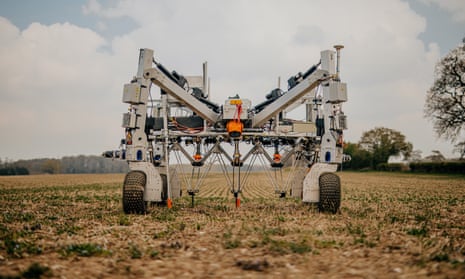
Gene editing crops to be colourful could aid weeding, say scientists
Creating visually distinctive plants likely to become important as more weed-like crops are grown for food
Genetically engineering crops to be colourful could help farmers produce food without pesticides, as it would make it easier to spot weeds, scientists have said.
This will be increasingly important as hardy, climate-resistant “weeds” are grown for food in the future, the authors have written in their report published in the journal Trends in Plant Science .
The lead author Michael Palmgren, a plant scientist from the University of Copenhagen, told the Guardian: “It can be modifications of hairs, leaf shape, light emitted at wavelength we cannot see. Anything could work on a large scale. The challenge of distinguishing a weed from a crop becomes imminent when we start breeding weeds.”
He said new crops were hard to distinguish from weeds, so it would be important to find a way to tell them apart. The paper suggests the crops’ genomes could be altered so they express pigments such as anthocyanins, which give blueberries their colour, or carotenoids, which make carrots orange.
“One example that we give in our opinion paper, fat hen ( Chenopodium album ), is grown for its nutritious seeds in India and Nepal and was a food source in Europe in the iron age – seeds are commonly found in the stomach of bog bodies,” said Palmgren.
“Today it is a robust and competitive weed in European fields, capable of producing significant crop losses. Some scientists say: why not improve fat hen to make it a new sustainable crop that does not need much care? If this becomes a reality, how to distinguish the improved fat hen from the wild, weedy fat hen? It is the same species and changes may first be observable after seeds have developed.”
Genetic science has helped find the genes responsible for the desirable traits that our ancestors selected for in crop plants, which means new crops with these traits can be bred much more rapidly using genetic engineering. Many wild plants are more tolerant to extreme weather and other climate-related impacts than current crop plants, so breeding them could help prevent food shortages as the climate breaks down.
However, these new crops are likely to resemble the weeds they are bred from, so to make weeding them easier without using pesticides, scientists suggest creating visually distinctive plants that robot weeders can easily differentiate from weeds.
“Distinguishing these new crops from their less productive and closely related wild plants could present tremendous challenges for weed control,” the researchers write. “Utilising gene editing to enhance their visual recognition by weeding robots could effectively address this issue.”
- Agriculture
- Climate crisis
Most viewed
NPR editor Uri Berliner resigns after bombshell expose reveals network’s pervasive left-wing bias
U ri Berliner, the veteran editor and reporter for National Public Radio who was suspended without pay after publishing a lengthy essay denouncing the outlet’s liberal bias, has resigned from the broadcaster.
“I am resigning from NPR, a great American institution where I have worked for 25 years,” Berliner wrote on his X social media account on Wednesday.
“I respect the integrity of my colleagues and wish for NPR to thrive and do important journalism.”
Berliner wrote that he “cannot work in a newsroom where I am disparaged by a new CEO whose divisive views confirm the very problems at NPR I cite in my Free Press essay.”
Berliner was referring to Katherine Maher, the chief executive at NPR who has come under fire for a series of “woke” social media posts in which she criticized Hillary Clinton for using the term “boy” and “girl” because it was “erasing language for non-binary people.”
Maher also appeared to justify looting In 2020 during the Black Lives Matter protests, saying it was “hard to be mad” about the destruction. In 2018, she wrote a post denouncing then-President Donald Trump as a “racist” before deleting it.
On Tuesday, NPR spokeswoman Isabel Lara said in a statement that Maher “was not working in journalism at the time and was exercising her First Amendment right to express herself like any other American citizen.”
Berliner, a Peabody Award-winning journalist, called out journalistic blind spots around major news events, including the origins of COVID-19, the war in Gaza and the Hunter Biden laptop, in an essay published last Tuesday on Bari Weiss’ online news site the Free Press.
In Berliner’s essay — titled “I’ve Been at NPR for 25 years. Here’s How We Lost America’s Trust” — Berliner said that among editorial staff at NPR’s Washington, DC, headquarters, he counted 87 registered Democrats and no Republicans.
He wrote that he presented these findings to his colleagues at a May 2021 all-hands editorial staff meeting.
“When I suggested we had a diversity problem with a score of 87 Democrats and zero Republicans, the response wasn’t hostile,” Berliner wrote. “It was worse. It was met with profound indifference.”
Maher, who took up the role as CEO of NPR in late March, responded to Berliner’s essay by claiming that the veteran journalist was being “profoundly disrespectful, hurtful, and demeaning” to his colleagues.
She accused Berliner of “questioning whether our people are serving our mission with integrity … based on little more than the recognition of their identity.”
Berliner also called out his bosses at NPR for their refusal to seriously cover the laptop story — which was exclusively broken by The Post.
The laptop contained emails showing that the son of President Biden was engaged in influence-peddling overseas — though NPR and other media outlets declined to aggressively cover the story in the run-up to the 2020 presidential election.
According to Berliner, senior editors at NPR feared that devoting airtime to the story would help Trump’s re-election chances just weeks before voters cast their ballots.
Berliner wrote that NPR had deteriorated into “an openly polemical news outlet serving a niche audience.”
“The laptop was newsworthy,” Berliner wrote. “But the timeless journalistic instinct of following a hot story lead was being squelched.”
Berliner also accused NPR of giving disproportionately more attention to allegations that Trump was colluding with the Russian government to win the 2016 presidential election — only to devote far less resources to Robert Mueller’s findings that there was insufficient evidence to bring criminal charges.
After the contents of the laptop proved to be authentic, NPR “could have fessed up to our misjudgment,” Berliner wrote.
“But, like Russia collusion [allegations against Trump that were debunked], we didn’t make the hard choice of transparency.”
Berliner also called out NPR for pushing other left-leaning causes, such as subjecting staffers to “unconscious bias training sessions” in the wake of the May 2020 death of George Floyd.
Employees were ordered to “start talking about race,” he said.
NPR journalists were also told to “keep up to date with current language and style guidance from journalism affinity groups” that were based on racial and ethnic identity, including “Marginalized Genders and Intersex People of Color” (MGIPOC), “NPR Noir” (black employees at NPR) and “Women, Gender-Expansive, and Transgender People in Technology Throughout Public Media.”
According to Berliner, if an NPR journalist’s language “differs from the diktats of those groups,” a “DEI Accountability Committee” would settle the dispute.
- Share full article
Advertisement
Supported by
NPR Suspends Editor Whose Essay Criticized the Broadcaster
Uri Berliner, a senior business editor at NPR, said the public radio network’s liberal bias had tainted its coverage of important stories.

By Benjamin Mullin
NPR has suspended Uri Berliner, the senior business editor who broke ranks and published an essay arguing that the nonprofit radio network had allowed liberal bias to affect its coverage.
Mr. Berliner was suspended by the network for five days, starting Friday, for violating the network’s policy against doing work outside the organization without first getting permission.
Mr. Berliner acknowledged his suspension in an interview with NPR on Monday , providing one of the network’s reporters with a copy of the written rebuke. In presenting the warning, NPR said Mr. Berliner had failed to clear his work for outside outlets, adding that he would be fired if he violated the policy again.
Mr. Berliner’s essay was published last week in The Free Press, a popular Substack publication.
He declined to comment about the suspension. NPR said it did not comment on personnel matters.
The revelation of Mr. Berliner’s punishment is the latest aftershock to rattle NPR since he published his essay. Employees at the public radio network were taken aback by Mr. Berliner’s public condemnation of the broadcaster, and several have said they no longer trust him because of his remarks. Mr. Berliner told The New York Times last week that he did not reach out to the network before publishing his essay.
After Mr. Berliner’s essay was published, NPR’s new chief executive, Katherine Maher, came under renewed scrutiny as conservative activists resurfaced a series of years-old social media posts criticizing former President Donald J. Trump and embracing progressive causes. One of the activists, Christopher Rufo, has pressured media organizations into covering controversies involving influential figures, such as the plagiarism allegations against Claudine Gay, the former Harvard president.
NPR said on Monday that Ms. Maher’s social media posts were written long before she was named chief executive of NPR, and that she was not working in the news industry at the time. NPR also said that while she managed the business side of the nonprofit, she was not involved in its editorial process. Ms. Maher said in a statement that “in America everyone is entitled to free speech as a private citizen.”
Several NPR employees have urged the network’s leaders to more forcefully renounce Mr. Berliner’s claims in his essay. Edith Chapin, NPR’s top editor, said in a statement last week that managers “strongly disagree with Uri’s assessment of the quality of our journalism,” adding that the network was “proud to stand behind” its work.
Some employees have begun to speak out. Tony Cavin, NPR’s managing editor for standards and practices, took issue with many of Mr. Berliner’s claims in an interview with The Times on Tuesday, saying Mr. Berliner’s essay mischaracterized NPR’s coverage of crucial stories.
Mr. Cavin said NPR’s coverage of Covid-19, one of the lines of reporting that Mr. Berliner criticized, was in step with reporting from other mainstream news organizations at the time. The coverage, he said, attributed the origins of the virus to a market in Wuhan, China. He also defended NPR’s coverage of Russian interference in the 2016 U.S. election, another area Mr. Berliner focused on, noting that Robert Mueller, the special counsel investigating the issue, concluded that Russian state actors had made attempts to sway the election.
Mr. Cavin also pointed out that NPR had no way to verify early articles about Hunter Biden’s laptop after the story broke but pursued follow-up stories examining the situation. Mr. Berliner wrote that NPR had “turned a blind eye” to the story about Mr. Biden’s laptop.
“To somehow think that we were driven by politics is both wrong and unfair,” Mr. Cavin said.
Benjamin Mullin reports on the major companies behind news and entertainment. Contact Ben securely on Signal at +1 530-961-3223 or email at [email protected] . More about Benjamin Mullin

An official website of the United States government
The .gov means it’s official. Federal government websites often end in .gov or .mil. Before sharing sensitive information, make sure you’re on a federal government site.
The site is secure. The https:// ensures that you are connecting to the official website and that any information you provide is encrypted and transmitted securely.
- Publications
- Account settings
Preview improvements coming to the PMC website in October 2024. Learn More or Try it out now .
- Advanced Search
- Journal List
- Children (Basel)

Utility of Genetic Testing from the Perspective of Parents/Caregivers: A Scoping Review
Robin z. hayeems.
1 Child Health Evaluative Sciences, The Hospital for Sick Children, Toronto, ON M5G 1X8, Canada; [email protected] (S.L.); [email protected] (D.A.); ac.owu@28ttahba (A.B.); [email protected] (W.J.U.)
2 Institute of Health Policy Management and Evaluation, The University of Toronto, Toronto, ON M5T 3M6, Canada
Stephanie Luca
Daniel assamad, ayushi bhatt.
3 Schulich School of Medicine and Dentistry, Western University, London, ON N6A 5C1, Canada
Wendy J. Ungar
Associated data.
All data generated or analysed during this study are included in this published article (and its supplementary information files ).
In genomics, perceived and personal utility have been proposed as constructs of value that include the subjective meanings and uses of genetic testing. Precisely what constitutes these constructs of utility and how they vary by stakeholder perspective remains unresolved. To advance methods for measuring the value of genetic testing in child health, we conducted a scoping review of the literature to characterize utility from the perspective of parents/caregivers. Peer reviewed literature that included empiric findings from parents/caregivers who received genetic test results for an index child and was written in English from 2016–2020 was included. Identified concepts of utility were coded according to Kohler’s construct of personal utility. Of 2142 abstracts screened, 33 met inclusion criteria. Studies reflected a range of genetic test types; the majority of testing was pursued for children with developmental or neurodevelopmental concerns. Coding resulted in 15 elements of utility that mapped to Kohler’s four domains of personal utility (affective, cognitive, behavioural and social) and one additional medical management domain. An adapted construct of utility for parents/caregivers may enable specific and standardized strategies for researchers to use to generate evidence of the post-test value of genetic testing. In turn, this will contribute to emerging methods for health technology assessment and policy decision making for genomics in child health.
1. Introduction
Anticipated benefits of existing and emerging genome diagnostics include definitive diagnosis and risk identification, enabling early intervention and a precision medicine approach to management that can potentially reduce morbidity and improve quality of life [ 1 , 2 ]. In addition to identifying genetic variants causally associated with a given indication for testing, genome-wide technologies have the capacity to identify variants associated with pre-symptomatic health risks and pharmaceutical sensitivities [ 2 , 3 , 4 ]. As such, applications of genome-wide technologies may be particularly beneficial in children since early diagnosis, risk identification and tailored intervention can result in lifelong benefit. Moreover, diagnosis and risk information generated for an index child may have significant health and non-health related implications for family members [ 5 ].
Defining and measuring the notion of benefit in the context of genome diagnostics requires the inclusion of metrics that extend beyond laboratory-based performance and incorporate patients’ perspectives [ 6 , 7 , 8 , 9 ]. This requirement aligns with a broader transformation in clinical research that has prioritized ascertaining patient and family experiences [ 10 ]. While a wide range of patient-reported outcome measures have been used in the genomics literature to reflect on patients’ preferences, experiences, and psychosocial responses to genetic testing [ 11 ], patient-oriented utility (i.e., personal or perceived utility) has emerged as a core construct that unifies many of these elements and reflects on the subjective informational value of genetic testing. Personal and perceived utility have been described as constructs of value that include the subjective health and non-health-related meanings and uses of genetic testing and/or a genetic diagnosis, such as increasing knowledge and feelings of control, and optimizing care for individuals and family members in the present and for the future. Some scholars assert that patient-oriented utility (be it ‘personal’ or ‘perceived’) is inclusive of unfavourable effects of genetic testing (i.e., risks related to privacy, discrimination, distress) [ 12 , 13 , 14 , 15 ], while others suggest unfavourable effects do not reflect this construct [ 16 , 17 ].
Most recently, Kohler et al. [ 16 ] conducted a systematic review of the literature and characterized the concept of personal utility as inclusive of affective, cognitive, behavioural, and social domains. Using a Delphi approach, Kohler et al. [ 17 ] established a list of 24 items that represent 14 elements of personal utility, grouped into four domains. Kohler’s construct emphasizes personal utility as a non-health related outcome measure, while others have characterized the value of genetic testing from patients’ perspectives more broadly to include health and clinical-management related impacts. Scheuner et al., [ 18 ] for example, engaged patient, clinician, researcher, administrator, and policy maker stakeholders in a modified Delphi process and identified health and medical management as important domains in characterizing the impact of genetic testing. Additional domains identified to be important by this panel included reproductive, diagnostic/prognostic, patient behavioural, patient psychosocial, and family-related. Similarly, from our earlier work with parents of children who received genetic testing, we learned that parents characterize the value of genetic testing as intrinsic in terms of the test’s ability to provide a much sought-after answer for their child’s condition, and as instrumental in terms of its ability to guide care, access health and social services, reduce stigma, make family planning decisions, and engage in proactive prevention strategies [ 15 , 19 , 20 ]. Finally, Bunnik [ 12 ] differentiates perceived and personal utility, emphasizing that personal utility is only achieved when (valid) genetic information is useful (i.e., instrumental) in guiding actions of a personal nature.
What constitutes patient-oriented utility is complex and multi-dimensional. Arguably, how the construct is defined also depends on the clinical context and patient/respondent population to whom it is offered [ 12 , 13 ]. In the pediatric context, generally speaking, measuring patient-reported outcomes is challenged by the fact that children are not typically cognitively capable of making assessments that require abstract thinking and proxy measures (whereby parents respond for their child) do not provide an authentic assessment of the child’s perspective [ 21 , 22 , 23 ]. In genomics, this measurement challenge is compounded by the fact that an intervention like a genetic test, when performed on an index child, has health and non-health related impacts that extend beyond the child, to parents and other family members. Even if a measurement tool could capture a child’s authentic perspective, it would likely provide a limited understanding of the impact of genetic testing on the family unit overall. As such, although parents/caregivers cannot serve as perfect proxies for their child’s views, they are well-positioned to provide a personal account of the utility of a genetic test for themselves and for the family unit, inclusive of the child. To provide an overview of the literature on utility from the parent/caregiver perspective and to inform the development of emerging value frameworks and health technology assessment methods for precision medicine, [ 8 ] we conducted a scoping review of the literature. Building on Kohler’s work [ 16 , 17 ], we focused specifically on parental/caregiver utility associated with receiving genetic test results.
2. Materials and Methods
2.1. search strategy.
This study complies with the “Preferred Reporting Items for Systematic reviews and Meta-Analysis extension for Scoping Reviews (PRISMA-ScR)” guidelines [ 24 ]. Papers that have been central to understanding the construct of patient-oriented utility in the genetics literature [ 12 , 14 , 15 , 16 , 17 , 18 , 19 , 20 ] were used to generate a preliminary list of key words. Search terms included: genetic testing OR genetic counseling AND patient reported outcome OR personal utility OR perceived utility OR personal value OR patient/caregiver preference. The full list of search terms is provided in Supplementary Material (Supplementary Figure S1: Search Strategy) . As described, patient-oriented utility can include health and non-health related outcomes of genetic testing that have either instrumental or intrinsic value to patients and families [ 12 , 13 , 14 , 15 , 16 , 17 ]. To ensure sufficient coverage of this construct after an initial search, this list was expanded and a full search strategy was developed in consultation with a medical librarian. The SCOPUS, MEDLINE, EMBASE and Web of Science databases were searched for English language articles published between 1 January 2016 and 31 December 2020; to capture the period of time that directly follows Kohler’s critical work in this area [ 16 , 17 ], enabling us to build on their findings.
2.2. Study Selection
Search results from all four databases were imported into an EndNote (X7) library. Duplicate articles were identified and removed using the Bramer method [ 25 ]. Conference publication titles were removed at the outset where possible. The abstracts of all remaining studies were screened by two authors according to the following inclusion criteria: (i) English language, published between 1 January 2016 and 31 December 2020, contained empirical evidence, peer-reviewed, (ii) study collected data after genetic test results were reported to patients/families, (iii) study design included parent/caregiver reported outcomes, experiences, preferences, or values related to results received from genetic testing (i.e., actual results, not hypothetical scenarios). The following were excluded: conference materials, editorials, consensus statements, practice guidelines, meta-analysis, systematic reviews, opinion or workgroup papers, case reports/series, discrete choice experiments, studies primarily related to diagnostic yield or gene discovery, hypothetical genetic testing, cost effectiveness, or outcome measure development or validation. If it was unclear from the abstract whether or not a study was eligible, it was included for full text review. Eligible studies published before 1 January 2016 were excluded since we anchored our approach to Kohler’s construct of personal utility [ 16 ]. Full text review of remaining articles ( n = 138) was conducted by two independent reviewers and followed the same inclusion criteria as above. Any differences in assessment of eligibility between the two reviewers were resolved by discussion and a third reviewer was consulted where necessary. The percent agreement between raters with regard to eligibility was determined.
2.3. Data Extraction and Synthesis
Two authors extracted the following characteristics from each study: PubMed ID, authors, publication year, study title, country of data collection, respondent type, International Classification of Diseases (ICD-11) disease category [ 26 ] for index case, genetic test type pursued, sample size, study design, utility-related outcomes, and utility related themes. Where more than one sample was described in an article, the larger sample was identified as the primary sample and the smaller sample was identified as the secondary sample. For example, some studies reported survey findings from the full sample as well as qualitative findings ascertained from a sub-sample of survey participants. Utility referred to parent/caregiver reported outcomes, experiences, preferences, or values related to the information available or received from genetic testing.
Organizing and synthesizing extracted outcomes and themes followed a multi-step process. First, outcomes and themes were extracted from the final eligible pool of studies. These outcomes and themes were assigned to domains of personal utility, as described by Kohler [ 17 ] (i.e., affective, cognitive, behavioral, social). An ‘other’ category was included to capture outcomes and themes that did not fall into Kohler’s domains of utility. The two authors who completed full text extractions reviewed domain assignments and resolved discrepancies that emerged through discussion. This mapping exercise endorsed the four domains of personal utility identified by Kohler et al., and identified a medical management domain similar to that identified by Scheuner’s value framework and related empiric work [ 12 , 13 , 14 , 15 , 18 ]. For each Kohler domain, outcomes and themes were mapped to pre-established elements and element concepts. Where our extracted outcomes and themes aligned with Kohler’s element concepts, we retained these concepts. Where new concepts emerged or where concepts warranted re-characterization to suit the parent/caregiver perspective, modifications were made. For the medical management domain, new elements and element concepts were defined [ 12 , 13 , 14 , 15 , 18 ].
2.4. Quality Appraisal
A quality appraisal of all studies was conducted. The QualSyst quality assessment criteria for qualitative, quantitative, and mixed methods studies were used ( Supplementary Table S1 : Quality appraisal scores) [ 27 ]. For the quantitative studies, 14 items (e.g., assessing study objective, methodology, analysis, conclusions) were scored depending on the degree to which the specific quality criteria were met (“criteria met” = 2, “criteria partially met” = 1, “criteria not met” = 0). Items not applicable to a particular study design were marked “n/a” and were excluded from the calculation of the summary score. A score was calculated for each article by summing the scores obtained across relevant items and dividing by the total possible score. Scores for the qualitative studies were calculated in a similar fashion based on the scoring of ten items. Since assigning “n/a” was not permitted for qualitative studies [ 27 ], the total possible score for each qualitative study was 20. For mixed methods studies, an additional five criteria from the Mixed Methods Appraisal Tool (MMAT) [ 28 ], assessing the integration of qualitative and quantitative methods and conclusions Supplementary Table S1 , were rated on the same 0 to 2 scale. Final scores for mixed methods studies were determined by adding the scores from the qualitative, quantitative, and mixed methods appraisal tools, divided by the total possible score [ 28 ]. Quality score averages and ranges were calculated Table 1 .
Study characteristics ( n = 33).
a Multiple characteristics may apply to a single paper; b the ICD-11 classification “Pregnancy, childbirth or the puerperium” was relabeled to “Prenatal or pre-implementation genetic testing” for the purpose of this review to more accurately describe the studies in this category; c other disease category = Endocrine, nutritional or metabolic diseases, diseases of the circulatory system, diseases of the visual system, visibly healthy, recessive and X-linked conditions in offspring; d prenatal genetic test type = Non-invasive prenatal testing (NIPT), pre-implantation genetic screening; e other genetic test type = Tumour sequencing or commercial publicly available. Abbreviations: FISH = fluorescence in situ hybridization.
3.1. Study Selection, Characteristics and Quality
Our comprehensive search yielded 4499 abstracts. Duplicates were removed and the remaining 2142 abstracts were screened for inclusion. After removing 2004 conference or literature review abstracts that did not meet inclusion criteria, 138 full-text papers were reviewed. Of these, 105 were excluded based on lack of empirical results, or study designs that focused on hypothetical response to genetic testing, pre-test perceptions of genetic testing, and/or non-parent/caregiver reported outcomes. Thirty-three [ 15 , 29 , 30 , 31 , 32 , 33 , 34 , 35 , 36 , 37 , 38 , 39 , 40 , 41 , 42 , 43 , 44 , 45 , 46 , 47 , 48 , 49 , 50 , 51 , 52 , 53 , 54 , 55 , 56 , 57 , 58 , 59 , 60 ] met all inclusion criteria Figure 1 . All full texts were reviewed for inclusion by two independent reviewers, with an inter-rater agreement of 83.4%. Absence of consistency between raters pertained to raters’ oversight of study details related to inclusion criteria.

PRISMA flow diagram.
The majority (55%) of the studies were conducted in the United States. The remaining studies were conducted in Europe (18%), Australia (15%), Canada (9%), and New Zealand (3%). Of the 33, 20 (61%) were qualitative, nine (27%) were quantitative, and four (12%) were mixed methods. In decreasing order, the most common genetic tests on which study participants reflected were chromosome microarray ( n = 16, 48.5%), whole exome sequencing ( n = 14, 42.4%), multi-gene panel ( n = 6, 18.2%), and karyotype ( n = 5, 15.2%). The 33 studies included a total of 3606 primary sample participants and 93 secondary sample participants. Respondent type included: parents/caregivers of minor patient (73%), prospective parents (i.e., prenatal testing context; 16%), caregivers of adult patient (8%), and other (3%). The most common clinical conditions under study were developmental anomalies (61%), behavioural or neurodevelopmental disorders (39%), prenatal or pre-implementation genetic testing (27%), and diseases of the nervous system (21%) ( Table 1 ). The complete list of included studies is provided in Supplementary Table S2 : Scoping review studies and assigned study number.
With respect to quality, quantitative studies reviewed scored the highest, with an average score of 82.9%, compared to qualitative (81.0%) and mixed methods (80.8%) studies. Almost all qualitative studies were given a perfect rating for study design; the reflexivity item (i.e., extent to which authors consider their own characteristics or worldview during data collection and analysis) scored the lowest. All quantitative studies had clear study objectives and design; the lowest scoring item related to controlling for confounding. Two of the four mixed methods studies did not address inconsistencies between qualitative and quantitative findings well. No studies were excluded based on their quality appraisal score Supplementary Table S1 .
3.2. Utility from Parents’/Caregivers’ Perspectives
The outcomes and themes identified in 33 studies that were specific to the receipt of results from genetic testing from parents’/caregivers’ perspectives aligned with Kohler’s four domains and 14 elements of utility. The outcomes and themes characterized as element concepts associated with each element of each domain are displayed in Table 2 . The element specific to “feelings of responsibility” (affective domain) was most commonly reported (72.7% of studies). The element specific to “knowledge of condition” (cognitive domain) was reported in 69.7% of studies and the elements of “reproductive autonomy” (behavioural domain) and “change in social support” (social domain) were reported in 60.9% of studies. In addition, we identified a management domain which included an element related to clinician-directed management activities and element concepts related to initiation of or alteration to medication use, ongoing diagnostic testing, surveillance and monitoring, and sub-specialist or community referrals. Clinician directed activities applied to both probands and family members. This domain of utility was identified in 48.5% of studies reviewed.
Elements of personal utility from the perspective of parents/caregivers.
Notes: Concepts in italics represent a new personal utility domain (i.e., medical management) and modifications to Kohler’s element concepts as per Kohler et al. 2017 [ 17 ]. Study numbers correspond to Supplementary Table S2 .
While Kohler’s four domains of personal utility were identified in the studies reviewed, our findings extend the definitions of some of Kohler’s domain elements to include aspects of utility that were germane to parents/caregivers. While these aspects may be implicit in the definitions of the existing elements in Kohler’s framework, our review prompted us to explicitly name these aspects as important parts of the construct of utility when thinking about it from parent/caregiver perspectives.
In the affective domain, where outcomes of genetic testing refer to an individual’s emotional state, [ 17 ] the studies reviewed suggest the addition of concepts to three affective elements. For ‘enhanced coping’, studies reviewed include peace of mind and relief and for ‘mental preparation’, studies reviewed include the concept of hope. For ‘feelings of responsibility,’ studies reviewed include the concepts of guilt, worry and sense of competence. In the cognitive domain, where outcomes refer to gains in knowledge or information from genetic testing, studies reviewed suggest the addition of concepts to each of the four elements. For ‘value of information’, studies reviewed emphasize the concept of closure and for ‘knowledge of condition’, studies include explicit knowledge related to diagnosis, prognosis, and recurrence risks. For ‘curiosity’, studies reviewed emphasize a need to satisfy curiosity and seek information. For ‘self-knowledge’, studies reviewed emphasize the importance of understanding the child (i.e., not oneself) with the medical condition.
In the behavioural domain, where outcomes represent practical uses of genomic information, studies reviewed suggest the addition of concepts to each of the three existing elements of utility. Regarding ‘ability for future planning’, studies reviewed include impact on career, finances and lifestyle for parents. Regarding ‘reproductive autonomy’, studies reviewed extend this element to include informing planning of future pregnancies not only for the index case but also for other family members. Regarding ‘communication’, while communication with family members is inclusive of all members, studies reviewed describe communication with the child as a distinct concept. Finally, in the social domain, where outcomes of genetic testing involve changes in social support or status on individual, familial, and societal levels, the only additional concept identified in the studies reviewed related to the ‘concern over discrimination’ element. Similar to Kohler’s systematic review [ 16 ], studies reviewed here indicated that privacy concerns warrant inclusion in this element.
4. Discussion
Adapting the construct of personal utility derived from Kohler’s systematic review [ 16 ] and Delphi’s study [ 17 ] for parents/caregivers, we identified 15 distinct elements of utility spanning five non-overlapping domains. While two elements (i.e., self-knowledge and feeling good for helping others) were identified in only three studies reviewed and one (i.e., spiritual well-being) was identified in only one, all other elements were identified in five or more independent studies, indicating that these elements comprise a good representation of the construct of utility, as perceived by parents/caregivers. Parents of children with developmental or neurodevelopmental indications for genetic testing represented the most common perspective captured. While most included studies focused on these clinical indications, a range of genetic test types (i.e., microarray, single gene, gene panel, exome sequencing) were considered by parent/caregiver respondents, including some prospective parents. As such, findings herein are not limited to the context of exome/genome sequencing but apply to a range of diagnostic genetic testing strategies.
Our findings map neatly onto four domains and 14 elements of personal utility identified by Kohler [ 16 , 17 ]. Within the 14 elements, our findings suggest that important modifications are warranted to characterize the construct of utility from parents’/caregivers’ perspectives. These include (i) the addition of the concepts of hope, guilt, worry, competence, peace of mind and relief to the affective domain, (ii) the addition of the concepts of closure, information seeking, knowledge related to diagnosis, prognosis, and recurrence risks and explicit attention to understanding the child to the cognitive domain, (iii) explicit differentiation of behavioural impacts of test results on parents, children, and other family members in the behavioural domain, and (iv) the inclusion of the concept of privacy in the social domain. While adding concepts related to peace of mind and privacy represent an adaptation to the elements defined in Kohler’s Delphi study [ 17 ], these concepts were identified in the systematic review [ 16 ] they conducted to inform the Delphi process.
A significant difference between Kohler’s characterization of personal utility and that defined by the studies reviewed herein is the addition of the medical management domain. In related literature about genetic test evaluation, medical management implications are more often described as a component of the clinical utility construct. While many have argued that medical management implications constitute health-related outcomes and fit within the construct of clinical utility, others consider medical management as an example of an indirect or intermediate non-health related outcome, since a medical management decision, unto itself, does not comprise a health outcome [ 12 , 13 , 61 , 62 , 63 , 64 , 65 , 66 , 67 , 68 , 69 ]. While the distinction between health and non-health related outcomes remains important to the task of defining and adjudicating the value of genetic testing [ 67 , 68 , 69 ], it is plausible that both health and non-health related outcomes can be perceived and experienced from both clinical and personal perspectives. There is justification for medical management implications, as an impact of genetic testing that sits at the boundary of health and non-health related outcomes, to be included in both personal and clinical utility constructs. Findings from the present review suggest that medical management implications are an important aspect of utility from parent/caregiver perspectives and warrant inclusion in the definition of this construct.
Several patient-reported outcome measures have been validated for use in genetics, many of which include elements of the construct of personal utility defined by Kohler et al. [ 16 , 17 ] as well as the modified construct defined herein. For example, McAllister et al. [ 70 ] and Grant et al. [ 71 ] developed and validated the Genetic Counseling Outcome Scale (GCOS-24) and the Genomics Outcomes Scale (GOS), respectively, to capture the impact of genetic counselling services on patient empowerment. While the dimensions of the GCOS (i.e., cognitive, decisional and behavioural control, emotional regulation, and hope) reflect on non-health-related benefits of genetic counselling and overlap with dimensions identified by Kohler and by our review, the measures are not specific to the impact of genetic testing, rather they are focused on the impact of genetic counselling services. Using a different theoretical underpinning than the GCOS and GOS, McConkie-Rosell et al. developed the Genome Empowerment Scale (GEmS) [ 72 ]. While this tool was designed to understand parents’ perspectives on the process of empowerment in the context of genetic testing, it is administered prior to testing and does not reflect on the actual impact of test results. Yusuf et al.’s measure of utility of biomarker testing (Perceived Utility of Biological (PUB) Testing) also focuses on the anticipated pre-test value of testing rather than the outcomes of testing [ 73 ]. The Feelings About genomiC Testing Results (FACToR) scale measures the post-test psychosocial impact of genomic findings [ 74 ] but includes only the affective and cognitive domains of the personal utility construct defined by Kohler et al. [ 16 , 17 ] Findings from the studies reviewed here suggest that the construct of utility, oriented around the post-test impact of genetic testing, from parents’/caregivers’ perspectives, can be differentiated from constructs contained in existing patient reported outcome measures in genomic medicine.
While findings from this work provide a more detailed characterization of the construct of utility from parents’/caregivers’ perspectives, we acknowledge specific limitations. First, study inclusion criteria were challenging to establish and apply given the absence of an agreed upon definition of the patient-oriented utility in the field of genomics to date. In part, however, the purpose of the review was to contribute to efforts to define this construct for parents/caregivers. Second, we excluded non-English studies, potentially leading to our omission of concepts relevant to utility for parents/caregivers. We also excluded discrete choice experiments, cost effectiveness studies, and outcome measure development or validation studies. While discrete choice experiments provide preference data and use choice sets that are typically derived from the lived experience of the target population, the data generated are technically hypothetical. Cost effectiveness and measurement development/validation studies may reflect patient-oriented utility but the intent of these studies is distinct from our focus on further characterizing the construct of utility from parents’/caregivers’ perspectives. Third, while we view our use of Kohler’s personal utility construct as a strength and as a reference point from which to tailor the construct of utility to parents/caregivers, it may have acted to constrain our thinking about the elements and concepts embedded in this construct, and may have limited the scope of the literature reviewed given that 2016 was our starting point. The exclusion of studies published in 2021 is a further limitation. Finally, we included studies of variable quality, potentially representing a reporting bias.
Patient-oriented utility has emerged in the genomics literature as a critical measure of value. While it is generally agreed that personal and perceived utility reflect on the subjective meaning an individual ascribes to genetic testing, consensus has not emerged on the precise definition of these and related constructs. For example, whether or not elements of these constructs are distinct from or overlapping with elements of clinical utility or with elements of psychosocial well-being remains contested [ 9 , 16 , 63 ]. In addition, some orient to personal and perceived utility as measures of anticipated (i.e., pre-test) value [ 72 , 73 ], some as a measure of actual (i.e., post-test) value [ 15 , 20 , 74 , 75 ], and some as a composite of both [ 16 , 17 , 70 , 71 ]. Finally, whether any one of these constructs can be applied to a range of clinical genetics settings and respondent types or whether tailored approaches are required to achieve face validity in a range of settings requires further consideration. Building on Kohler’s evidence-informed approach to define specific elements of personal utility [ 16 , 17 ], we offer a modified version of utility that attends to the parent/caregiver perspective.
The field of genomics presents a multitude of challenges for assessing the value of emerging technologies through formal health technology assessment [ 8 , 67 , 68 , 69 ] and for health technology assessment in child health in particular [ 76 ]. The construct of patient-oriented utility is gaining prominence in aspects of health technology assessment related to assessing patient preferences as well as the consideration of ethical, legal and social implications [ 77 ]. Refining the construct of utility to suit the parent/caregiver perspective presented in this review and developing strategies in the future to operationalize the measurement of parent/caregiver utility will contribute to methodological innovation in this field and a richer evidence base from which informed policy decisions about technology adoption can be made.
Acknowledgments
We acknowledge Viji Venkataramanan’s contribution to data extraction.
Supplementary Materials
The following are available online at https://www.mdpi.com/article/10.3390/children8040259/s1 , Figure S1: Search strategy, Table S1: Quality appraisal scores, Table S2: Scoping review studies and assigned study number.
Author Contributions
Conceptualization: R.Z.H.; Software: D.A., A.B.; Validation: R.Z.H., W.J.U.; Formal analysis: R.Z.H., S.L., D.A., A.B.; Investigation: S.L., D.A., A.B.; Resources: R.Z.H.; Data curation: S.L., D.A., A.B.; Writing original draft preparation: R.Z.H.; Writing review and editing: R.Z.H., S.L., D.A., A.B., W.J.U.; Visualization: S.L., D.A., A.B.; Supervision: R.Z.H., S.L., W.J.U.; Project administration: S.L; All authors have read and agreed to the published version of the manuscript.
This research received no external funding.
Institutional Review Board Statement
Not applicable.
Informed Consent Statement
Data availability statement, conflicts of interest.
The authors declare no conflict of interest.
Publisher’s Note: MDPI stays neutral with regard to jurisdictional claims in published maps and institutional affiliations.
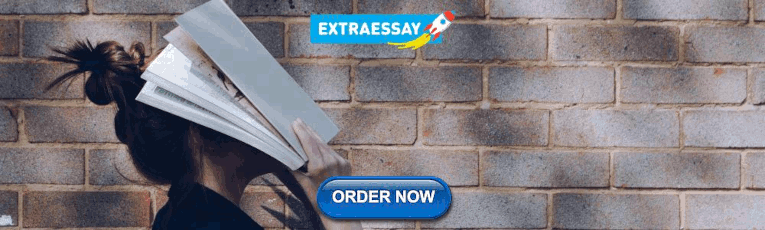
IMAGES
VIDEO
COMMENTS
One of the fundamental areas of this development is genetic research. Thus, the study of genetics makes it possible to solve pressing issues related to medicine, forensics, and security. This essay aims to discuss the public importance of supporting genetic research. We will write a custom essay on your topic.
Below, the benefits of genetic testing as a preventative healthcare measure are outlined. Genomics experts worldwide released a paper highlighting advances in rare disease, cancer, population health and carrier screening at the 10th Annual Individualizing Medicine Conference on Saturday, Oct. 9. "The use cases we explored in the paper, 'The ...
Applied Clinical Genomics is the application of genetic information to the clinical setting, including improved diagnosis of disease and tailored treatment efficacy and safety. By discovering and defining the genes that underlie susceptibility to disorders, genetic information can be used to identify and better define those genes that play ...
Genomic research has evolved from seeking to understand the fundamentals of the human genetic code to examining the ways in which this code varies among people, and then applying this knowledge to ...
Advances in genetics and genomics are transforming medical practice, resulting in a dramatic growth of genetic testing in the health care system. The rapid development of new technologies, however, has also brought challenges, including the need for rigorous evaluation of the validity and utility of genetic tests, questions regarding the best ways to incorporate them into medical practice, and ...
Between 2014 and 2019, 301 of 1,281 patients pursued predictive genetic testing at Mayo's Predictive Genomics Clinic, where diagnosis and treatment are individually tailored. ... "Genetic counseling is an important part of predictive testing by helping patients understand the results and their options for preventive care." Learn more.
Introduction. With the rapid advances in genomic technologies during the past decades, genetic testing has become widely used in clinical practice for identification of individuals at increased risk for inherited conditions [].Cancer is the most common genetic disease; and genetic testing allows identification of high-risk individuals who may benefit from interventions that mitigate risk and ...
The goal of genetic testing is to identify if a person has a mutation linked to a specific disease. For some conditions, there is a known gene mutation that can be identified to diagnose the disease (e.g. Huntington's disease results from a mutation of the HTT gene). There are several methods of genetic testing currently available.
Genetic Testing. Genetic tests determine variations in genes. They may be used to help guide medical decisions, identify individuals, or provide information about how your body works. Genetic test results may encourage you to be proactive about lifestyle changes that may help manage health risks. A genetic marker is a DNA sequence with a known ...
Genetic testing is becoming a much more common practice in medicine today. This presents a unique set of challenges for medical professionals in virtually all specialties. The practical aspects of determining which test to order, and in interpreting the result accurately in the context of the family history, can be difficult. Additionally, the ethical conundrums that frequently present ...
Abstract. In celebration of the 20th anniversary of Nature Reviews Genetics, we asked 12 leading researchers to reflect on the key challenges and opportunities faced by the field of genetics and ...
Even when genetic testing is taken, the importance of medical advice should not be underestimated. Medical professionals are better informed due to their experience and knowledge and are better aware of the various options available to the customers. Genetic testing may have consequences that extend far beyond the individuals.
4 Reasons Why the Study of Genetics is Important. 1. Studying Genetics Gives an Understanding of Where We've Been. Studying genetics and its history provides the foundation for all future advancements. Knowing where genetic science has come from and the hurdles overcome by research helps students find their place in the greater story of genes.
The first limitation is that gender was not well balanced in all the selected studies and was not investigated as a factor that could influence the psycho-behavioral impact of genetic testing. We exclude papers on breast cancer (which investigated women samples), Li-Fraumeni Syndrome (LFS) (where men and female were equally balanced and female ...
Genetics forms one of the central pillars of biology and overlaps with many other areas, such as agriculture, medicine, and biotechnology. Since the dawn of civilization, humankind has recognized the influence of heredity and applied its principles to the improvement of cultivated crops and domestic animals. A Babylonian tablet more than 6,000 ...
Although 65% of the probands identified in these papers were male, nearly two‐thirds (65%) of the affected relatives identified through genetic testing were female. ... Education on the importance of genetic testing for rare genetic diseases may also be needed for general practice physicians, depending on the region/country, to overcome ...
It is therefore important to increase awareness among the medical community about the ethical dilemmas that regularly occur in relation to genetic testing, and the ways in which these can be managed. Findings from our study indicate that health professionals who regularly provide genetic tests are likely to encounter a range of ethical dilemmas.
Genes are composed of a substance called deoxyribonucleic acid, or DNA. DNA contains the biological instructions that allow for the development, growth, and reproduction of life. Chromosomes ...
NPR is defending its journalism and integrity after a senior editor wrote an essay accusing it of losing the public's trust. NPR's top news executive defended its journalism and its commitment to ...
Helena Horton Environment reporter. Wed 17 Apr 2024 11.00 EDT. Genetically engineering crops to be colourful could help farmers produce food without pesticides, as it would make it easier to spot ...
Tell me more about my genes. A person has two copies of each gene, one from the mother and one from the father. Genes carry instructions that tell your cells how to work and grow. Cells are the building blocks of the body. Every part of your body is made up of billions of cells working together. Genes are arranged in structures called chromosomes.
Uri Berliner, the veteran editor and reporter for National Public Radio who was suspended without pay after publishing a lengthy essay denouncing the outlet's liberal bias, has resigned from the ...
April 16, 2024. NPR has suspended Uri Berliner, the senior business editor who broke ranks and published an essay arguing that the nonprofit radio network had allowed liberal bias to affect its ...
2.1. Search Strategy. This study complies with the "Preferred Reporting Items for Systematic reviews and Meta-Analysis extension for Scoping Reviews (PRISMA-ScR)" guidelines [].Papers that have been central to understanding the construct of patient-oriented utility in the genetics literature [12,14,15,16,17,18,19,20] were used to generate a preliminary list of key words.
A number of sovereign debt restructurings over the past three years faced significant delays but the cases are now moving forward. These delays slowed access of countries to much needed Fund financial support, and alongside creditors' efforts the Fund had to find ways forward. With significant experience now gleaned from recent restructuring cases, it is important to extract the lessons for ...