- Português Br
- Journalist Pass
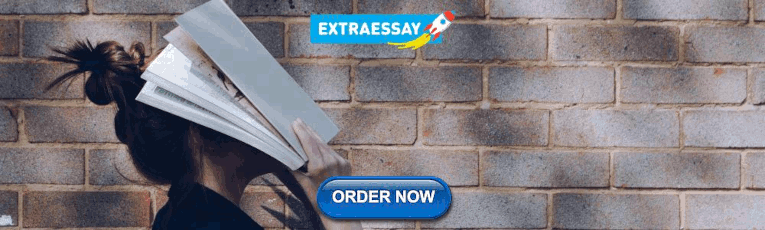
Mayo Clinic Minute: Is Alzheimer’s Type 3 diabetes?
Dennis Douda
Share this:
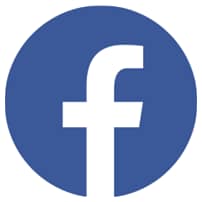
Are some cases of Alzheimer's disease triggered by a form of diabetes in the brain? Perhaps they are, according to researchers. Mayo Clinic's campuses in Rochester, Minnesota, and Jacksonville, Florida, recently participated in a multi-institution clinical study, testing whether a new insulin nasal spray can improve Alzheimer’s symptoms.
“This study has furthered our understanding of the gene that is the strongest genetic risk factor known for Alzheimer’s disease,” says Dr. Guojun Bu , a Mayo Clinic neuroscientist. "About 20 percent of the human population carries this riskier form of [the gene] APOE, called the E4," says Dr. Bu. It's believed that more than 50 percent of Alzheimer’s cases can be linked to APOE4, according to the study, which was published in Neuron .
Watch: The Mayo Clinic Minute
Journalists: A broadcast-quality video pkg (1:00) is in the downloads. Read the script .
It's an accepted fact that people with Type 2 diabetes have a higher risk of Alzheimer's disease. One reason may be reduced blood flow to the brain because of damaged blood vessels, Dr. Bu explains. "And, therefore, the supply of essential nutrients to the brain is also impaired."
Dr. Bu has found genetics may also be to blame. A variant of the so-called Alzheimer’s gene, APOE4, seems to interfere with brain cells' ability to use insulin, which may eventually cause the cells to starve and die. Unofficially, it's called Type 3 diabetes. "What it refers [to] is that their brain's insulin utilization or signaling is not functioning. Their risk of developing Alzheimer’s disease is about 10 to 15 times higher."
Researchers wondered if it is diabetes of the brain, could insulin delivered in an intranasal mist help patients? The results of a phase 2 clinical trial have raised hope. " The outcome is very positive. The patient's cognitive decline is slowed, if not improved." The multicenter research study will now expand into a broader phase 3 trial, with the backing of the National Institutes of Health.
- Breathing Easier After Minimally Invasive Heart Surgery Mayo Clinic implements Epic at Mayo Clinic Health System sites in Minnesota
Related Articles
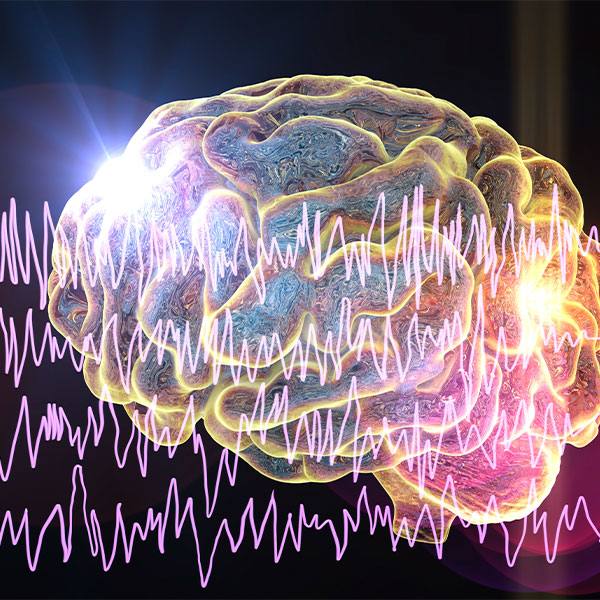
An official website of the United States government
Official websites use .gov A .gov website belongs to an official government organization in the United States.
Secure .gov websites use HTTPS A lock ( Lock Locked padlock icon ) or https:// means you've safely connected to the .gov website. Share sensitive information only on official, secure websites.
- Publications
- Account settings
- Advanced Search
- Journal List

The Role of Glucagon-Like Peptide 1 (GLP1) in Type 3 Diabetes: GLP-1 Controls Insulin Resistance, Neuroinflammation and Neurogenesis in the Brain
Choon sang bae, juhyun song.
- Author information
- Article notes
- Copyright and License information
Correspondence: [email protected] ; Tel.: +82-61-379-2706; Fax: +82-61-375-5834
Received 2017 Oct 30; Accepted 2017 Nov 20; Collection date 2017 Nov.
Licensee MDPI, Basel, Switzerland. This article is an open access article distributed under the terms and conditions of the Creative Commons Attribution (CC BY) license ( http://creativecommons.org/licenses/by/4.0/ ).
Alzheimer’s disease (AD), characterized by the aggregation of amyloid-β (Aβ) protein and neuroinflammation, is the most common neurodegenerative disease globally. Previous studies have reported that some AD patients show impaired glucose utilization in brain, leading to cognitive decline. Recently, diabetes-induced dementia has been called “type 3 diabetes”, based on features in common with those of type 2 diabetes and the progression of AD. Impaired glucose uptake and insulin resistance in the brain are important issues in type 3 diabetes, because these problems ultimately aggravate memory dysfunction in the brain. Glucagon-like peptide 1 (GLP-1) has been known to act as a critical controller of the glucose metabolism. Several studies have demonstrated that GLP-1 alleviates learning and memory dysfunction by enhancing the regulation of glucose in the AD brain. However, the specific actions of GLP-1 in the AD brain are not fully understood. Here, we review evidences related to the role of GLP-1 in type 3 diabetes.
Keywords: glucagon like peptide 1 (GLP-1), type 3 diabetes, diabetes-induced dementia, Alzheimer’s disease (AD), insulin resistance, Amyloid beta (Aβ)
1. Introduction
Alzheimer’s disease (AD) as an age-related neurodegenerative disorder is not well understood in terms of etiology, even though it was first described over 100 years ago [ 1 ]. AD is characterized by extracellular accumulation of aggregated amyloid-β (Aβ) protein, intracellular accumulation of hyper-phosphorylated tau protein, neuroinflammation, and a reduction in cerebral glucose consumption [ 2 ]. Recent studies have demonstrated that AD has a pathophysiological relationship with type 2 diabetes mellitus (T2DM), in that both involve impairment of insulin signaling and glucose metabolism [ 3 ]. Epidemiological studies have indicated that T2DM increases the risk of AD [ 4 , 5 ]. The brain has been known to regulate body energy and control food intake and body weight [ 6 , 7 ]. Additionally, the brain consumes glucose at a high rate, and uses it for propagation of action potentials and maintenance of the membrane potentials required for neuronal transmission [ 8 , 9 ]. AD patients show decreased glucose utilization in brain areas that are directly related to cognitive functions, including the hippocampus and cerebral cortex [ 10 ]. According to several studies, the deregulation of glucose metabolism in AD can be controlled by the administration of a hormone known as a potent regulator of glucose homeostasis [ 11 ] and of food intake [ 12 ], glucagon-like peptide 1 (GLP-1) [ 13 ]. The fact that administration of this peptide improves cognitive decline in patients with AD, as well as in AD mouse model [ 14 , 15 ] suggests that deregulation of glucose in the brain is a crucial issue in the onset and progression of AD [ 4 , 5 , 16 , 17 , 18 ]. Here, we review recent evidence concerning the role of GLP-1 in diabetes-induced dementia. We highlight the importance of GLP-1 in the onset and progression of diabetic AD, sometimes referred to as type 3 diabetes.
2. Diabetes Induced Dementia as the Type 3 Diabetes
Recent studies have demonstrated that patients with T2DM and metabolic syndrome have elevated risk for vascular dementia and AD [ 19 , 20 ]. Other studies have reported aberrant cerebral insulin homeostasis, which is called insulin resistance, in AD patients [ 21 , 22 ]. In the CNS, insulin is synthesized in neurons such as pyramidal and granule cells in the cerebral cortex and hippocampus [ 23 , 24 ]. Pancreatic insulin transported in small amounts across the blood–brain barrier (BBB) could also influence brain function [ 25 , 26 ]. Insulin growth factor-1 (IGF-1) and its receptor (IGF-1R) can be observed in the brain and have been related to the control of neurogenesis and synaptogenesis [ 27 , 28 ]. Deregulation of brain insulin signaling and IGF-1 signaling affects insulin resistance, energy metabolism, and lipid metabolism and results in pathological changes in the central nervous system (CNS) [ 29 , 30 , 31 , 32 ]. According to several studies, insulin and IGF-1 resistance can be detected in the brains of AD patients [ 29 ], but the relationship between insulin resistance and brain dysfunction remains unclear [ 33 ]. Recently, the relationship between brain insulin/IGF-1 signaling impairment and AD has been dubbed type 3 diabetes [ 34 ]. Further study of the mechanisms involved in the onset and progression of type 3 diabetes is necessary to improve our understanding of its pathology type 3 diabetes.
3. Glucagon-Like Peptide 1 (GLP1)
GLP-1 is an endogenous incretin hormone of 30-amino acids, produced by enteroendocrine L-cells, that influences food ingestion [ 35 , 36 ], enhances glucose-induced insulin secretion from pancreatic islets [ 37 ], and can act as a neuropeptide when released in the brain [ 38 ]. GLP-1 receptors (GLP-1R) exist widely throughout the brain, in areas including the hypothalamus, thalamus, hippocampus, cortex, and brainstem nucleus [ 39 , 40 , 41 ]. GLP-1 and other GLP-1 analogues can cross the BBB [ 42 , 43 ]. Because GLP-1 and its receptors exist in both the CNS and peripheral tissues, the effect of GLP-1 on energy metabolism is mediated by both the CNS and the peripheral nervous system (PNS) [ 11 , 44 , 45 ]. Moreover, GLP-1 is synthesized by neurons within the nucleus of the solitary tract [ 46 , 47 ]. These neurons have long projections to hypothalamic, thalamic, and cortical brain areas [ 48 ]. GLP-1 contributes to glycemic homeostasis and GLP1R agonists such as exendin-4, liraglutide, and lixisenatide have been approved to treat T2DM [ 49 , 50 ]. Furthermore, GLP-1 increases the spontaneous activity of neurons in the hippocampal CA1 region and promotes excitatory synaptic transmission in the hippocampus [ 51 ]. GLP-1 receptor knockout mice show decreased memory retention in the Morris water maze task, and the administration of GLP-1 agonists leads to improvement in learning and memory [ 52 ]. Here, given that GLP-1 could regulate glucose metabolism and potentially be used for treatment of T2DM [ 44 , 49 ], we focused on the role of GLP-1 in type 3 diabetes, highlighting the therapeutic importance of GLP-1 in diabetes-induced dementia.
4. The Effect of GLP-1 in Type 3 Diabetes: GLP-1 Attenuates Neuroinflammation and Improves Neurogenesis and Insulin Sensitivity in AD
One study suggested that GLP-1 mimetic drugs have neuroprotective, neurotrophic, and anti-inflammatory effects, which play a role in retardation of AD progression [ 14 ]. Another study demonstrated that liraglutide, a GLP-1 receptor agonist, can alleviate spatial memory dysfunction and neuroinflammation that leads to cognitive impairment [ 53 ]. GLP1 has been shown to act as a growth factor in the brain and promote neurite growth [ 54 ]. GLP-1 receptor activators stimulate the differentiation of neuronal stem cells in a manner similar to nerve growth factor, so it may inhibit brain atrophy in AD patients [ 55 ]. Additionally, GLP1 receptor agonists such as liraglutide and exendin-4 attenuate endogenous levels of amyloid beta in the brain and prevent amyloid plaque accumulation in the AD brain [ 42 , 53 ]. Furthermore, stimulating glucose metabolism in AD patients through the administration of GLP-1 markedly improves cognitive dysfunction in the AD brain [ 56 , 57 ]. In APP/PS1 mice (a mouse model of AD) brain, liraglutide and GLP-1 increase long-term potention (LTP) [ 42 , 58 ] and increase synaptic plasticity [ 41 , 55 , 59 ]. Moreover, GLP-1 has been found to improve insulin sensitivity [ 60 , 61 ] and control energy metabolism [ 62 , 63 ]. Recent studies reported that GLP-1 could attenuate brain insulin resistance by decreasing c-Jun N-terminal kinase (JNK) signaling and increasing the expression of the B-cell lymphoma 2 gene ( Bcl2 ) in the T2DM mouse [ 64 ]. One study demonstrated that liraglutide treatment in an AD mouse model triggers the activation of microglia in the brain [ 42 ]. Neurogenesis, the generation of new neurons from neuronal progenitor stem cells [ 65 , 66 ], occurs in the subventricular zone (SVZ) of the lateral ventricles and the subgranular zone of the hippocampal [ 67 , 68 ]. According to previous results, adult neurogenesis is linked to memory function and the facilitation of LTP [ 69 , 70 ]. In the AD brain, a decrease in neurogenesis is commonly observed and aggravates the disease pathology [ 41 , 71 ]. Several studies found that GLP-1 receptor agonists increase the proliferation of neural progenitor cells [ 41 ] and increase neurogenesis in the dentate gyrus of the hippocampus [ 43 ]. Earlier studies reported the impaired proliferation of neural stem cell in the AD mouse model [ 66 , 72 ] and that GLP-1 and analogues of GLP-1 can promote neural stem cell proliferation in the brain [ 73 , 74 ]. GLP-1 receptor activates neurogenesis in hippocampus through mitogen activated protein kinases (MAPK) [ 75 ], leading to enhancement of learning and memory [ 75 , 76 , 77 ]. Collectively, GLP-1 could attenuate neuroinflammation and enhance neurogenesis and insulin resistance in diabetes-induced dementia, also known as type 3 diabetes.
5. Conclusions
Summing up, we suggest that GLP-1 is a good candidate for improving cognitive dysfunction in diabetes-induced dementia. First, GLP-1 could attenuate the inflammatory responses in brain caused by amyloid beta (Aβ)-induced oxidative stress. GLP-1 could regulate the activation of microglia and protect neurons against oxidative stress. Second, GLP-1 could promote neurogenesis in AD brain. This means that GLP-1 could stimulate the generation of new neurons to replace damaged neurons in the AD brain. Finally, GLP-1 can alleviate insulin resistance in the AD brain, suggesting that impaired glucose metabolism and insulin resistance leads to severe memory dysfunction. To conclude, our study highlights that manipulation of GLP-1 may be an effective therapy for improving AD-like pathology in diabetes-induced dementia, also known as type 3 diabetes.
Acknowledgments
This study was supported by the Brain Research Program through the National Research Foundation of Korea funded by a grant from 2016R1D1A1B03930394.
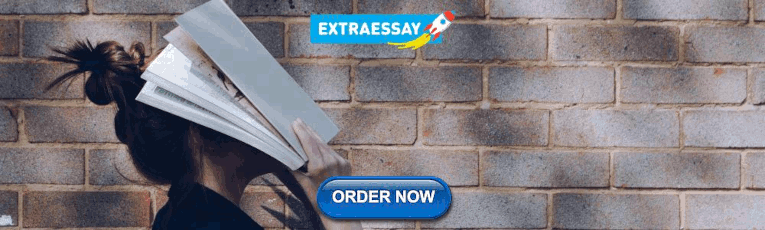
Author Contributions
Juhyun Song contributed to writing the preliminary draft of this manuscript and revised the manuscript. Choon Sang Bae contributed to writing the draft and revising manuscript as a whole.
Conflicts of Interest
The authors declare no conflict of interest.
- 1. Querfurth H.W., LaFerla F.M. Alzheimer’s disease. N. Engl. J. Med. 2010;362:329–344. doi: 10.1056/NEJMra0909142. [ DOI ] [ PubMed ] [ Google Scholar ]
- 2. LaFerla F.M., Green K.N. Animal models of Alzheimer disease. Cold Spring Harb. Perspect. Med. 2012;2:a006320. doi: 10.1101/cshperspect.a006320. [ DOI ] [ PMC free article ] [ PubMed ] [ Google Scholar ]
- 3. Akter K., Lanza E.A., Martin S.A., Myronyuk N., Rua M., Raffa R.B. Diabetes mellitus and Alzheimer’s disease: Shared pathology and treatment? Br. J. Clin. Pharmacol. 2011;71:365–376. doi: 10.1111/j.1365-2125.2010.03830.x. [ DOI ] [ PMC free article ] [ PubMed ] [ Google Scholar ]
- 4. Baglietto-Vargas D., Shi J., Yaeger D.M., Ager R., LaFerla F.M. Diabetes and Alzheimer’s disease crosstalk. Neurosci. Biobehav. Rev. 2016;64:272–287. doi: 10.1016/j.neubiorev.2016.03.005. [ DOI ] [ PubMed ] [ Google Scholar ]
- 5. Mamelak M. Energy and the Alzheimer brain. Neurosci. Biobehav. Rev. 2017;75:297–313. doi: 10.1016/j.neubiorev.2017.02.001. [ DOI ] [ PubMed ] [ Google Scholar ]
- 6. Roh E., Song D.K., Kim M.S. Emerging role of the brain in the homeostatic regulation of energy and glucose metabolism. Exp. Mol. Med. 2016;48:e216. doi: 10.1038/emm.2016.4. [ DOI ] [ PMC free article ] [ PubMed ] [ Google Scholar ]
- 7. Roh E., Kim M.S. Brain Regulation of Energy Metabolism. Endocrinol. Metab. 2016;31:519–524. doi: 10.3803/EnM.2016.31.4.519. [ DOI ] [ PMC free article ] [ PubMed ] [ Google Scholar ]
- 8. Attwell D., Laughlin S.B. An energy budget for signaling in the grey matter of the brain. J. Cereb. Blood Flow Metab. 2001;21:1133–1145. doi: 10.1097/00004647-200110000-00001. [ DOI ] [ PubMed ] [ Google Scholar ]
- 9. Magistretti P.J., Pellerin L. Metabolic coupling during activation. A cellular view. Adv. Exp. Med. Biol. 1997;413:161–166. doi: 10.1007/978-1-4899-0056-2_18. [ DOI ] [ PubMed ] [ Google Scholar ]
- 10. Doraiswamy P.M., Sperling R.A., Coleman R.E., Johnson K.A., Reiman E.M., Davis M.D., Grundman M., Sabbagh M.N., Sadowsky C.H., Fleisher A.S., et al. Amyloid-beta assessed by florbetapir F 18 PET and 18-month cognitive decline: A multicenter study. Neurology. 2012;79:1636–1644. doi: 10.1212/WNL.0b013e3182661f74. [ DOI ] [ PMC free article ] [ PubMed ] [ Google Scholar ]
- 11. Holst J.J. The physiology of glucagon-like peptide 1. Physiol. Rev. 2007;87:1409–1439. doi: 10.1152/physrev.00034.2006. [ DOI ] [ PubMed ] [ Google Scholar ]
- 12. Barrera J.G., Jones K.R., Herman J.P., D’Alessio D.A., Woods S.C., Seeley R.J. Hyperphagia and increased fat accumulation in two models of chronic CNS glucagon-like peptide-1 loss of function. J. Neurosci. 2011;31:3904–3913. doi: 10.1523/JNEUROSCI.2212-10.2011. [ DOI ] [ PMC free article ] [ PubMed ] [ Google Scholar ]
- 13. Sherwood V. WNT signaling: An emerging mediator of cancer cell metabolism? Mol. Cell. Biol. 2015;35:2–10. doi: 10.1128/MCB.00992-14. [ DOI ] [ PMC free article ] [ PubMed ] [ Google Scholar ]
- 14. Holscher C. Central effects of GLP-1: New opportunities for treatments of neurodegenerative diseases. J. Endocrinol. 2014;221:T31–T41. doi: 10.1530/JOE-13-0221. [ DOI ] [ PubMed ] [ Google Scholar ]
- 15. Talbot K., Wang H.Y. The nature, significance, and glucagon-like peptide-1 analog treatment of brain insulin resistance in Alzheimer’s disease. Alzheimers Dement. 2014;10:S12–S25. doi: 10.1016/j.jalz.2013.12.007. [ DOI ] [ PMC free article ] [ PubMed ] [ Google Scholar ]
- 16. Peng S., Eidelberg D., Ma Y. Brain network markers of abnormal cerebral glucose metabolism and blood flow in Parkinson’s disease. Neurosci. Bull. 2014;30:823–837. doi: 10.1007/s12264-014-1472-x. [ DOI ] [ PMC free article ] [ PubMed ] [ Google Scholar ]
- 17. Berti V., Mosconi L., Pupi A. Brain: Normal variations and benign findings in fluorodeoxyglucose-PET/computed tomography imaging. PET Clin. 2014;9:129–140. doi: 10.1016/j.cpet.2013.10.006. [ DOI ] [ PMC free article ] [ PubMed ] [ Google Scholar ]
- 18. Carpenter K.L., Jalloh I., Gallagher C.N., Grice P., Howe D.J., Mason A., Timofeev I., Helmy A., Murphy M.P., Menon D.K., et al. 13C-labelled microdialysis studies of cerebral metabolism in TBI patients. Eur. J. Pharm. Sci. 2014;57:87–97. doi: 10.1016/j.ejps.2013.12.012. [ DOI ] [ PMC free article ] [ PubMed ] [ Google Scholar ]
- 19. Tolppanen A.M., Lavikainen P., Solomon A., Kivipelto M., Uusitupa M., Soininen H., Hartikainen S. History of medically treated diabetes and risk of Alzheimer disease in a nationwide case-control study. Diabetes Care. 2013;36:2015–2019. doi: 10.2337/dc12-1287. [ DOI ] [ PMC free article ] [ PubMed ] [ Google Scholar ]
- 20. Biessels G.J., Strachan M.W., Visseren F.L., Kappelle L.J., Whitmer R.A. Dementia and cognitive decline in type 2 diabetes and prediabetic stages: Towards targeted interventions. Lancet Diabetes Endocrinol. 2014;2:246–255. doi: 10.1016/S2213-8587(13)70088-3. [ DOI ] [ PubMed ] [ Google Scholar ]
- 21. Butterfield D.A., Di Domenico F., Barone E. Elevated risk of type 2 diabetes for development of Alzheimer disease: A key role for oxidative stress in brain. Biochim. Biophys. Acta. 2014;1842:1693–1706. doi: 10.1016/j.bbadis.2014.06.010. [ DOI ] [ PMC free article ] [ PubMed ] [ Google Scholar ]
- 22. Bedse G., Di Domenico F., Serviddio G., Cassano T. Aberrant insulin signaling in Alzheimer’s disease: Current knowledge. Front. Neurosci. 2015;9:204. doi: 10.3389/fnins.2015.00204. [ DOI ] [ PMC free article ] [ PubMed ] [ Google Scholar ]
- 23. Devaskar S.U., Giddings S.J., Rajakumar P.A., Carnaghi L.R., Menon R.K., Zahm D.S. Insulin gene expression and insulin synthesis in mammalian neuronal cells. J. Biol. Chem. 1994;269:8445–8454. [ PubMed ] [ Google Scholar ]
- 24. Kuwabara T., Kagalwala M.N., Onuma Y., Ito Y., Warashina M., Terashima K., Sanosaka T., Nakashima K., Gage F.H., Asashima M. Insulin biosynthesis in neuronal progenitors derived from adult hippocampus and the olfactory bulb. EMBO Mol. Med. 2011;3:742–754. doi: 10.1002/emmm.201100177. [ DOI ] [ PMC free article ] [ PubMed ] [ Google Scholar ]
- 25. Banks W.A., Owen J.B., Erickson M.A. Insulin in the brain: There and back again. Pharm. Ther. 2012;136:82–93. doi: 10.1016/j.pharmthera.2012.07.006. [ DOI ] [ PMC free article ] [ PubMed ] [ Google Scholar ]
- 26. Le Roith D., Hendricks S.A., Lesniak M.A., Rishi S., Becker K.L., Havrankova J., Rosenzweig J.L., Brownstein M.J., Roth J. Insulin in brain and other extrapancreatic tissues of vertebrates and nonvertebrates. Adv. Metab. Disord. 1983;10:303–340. doi: 10.1016/b978-0-12-027310-2.50017-7. [ DOI ] [ PubMed ] [ Google Scholar ]
- 27. Kar S., Chabot J.G., Quirion R. Quantitative autoradiographic localization of [125I] insulin-like growth factor I, [125I] insulin-like growth factor II, and [125I] insulin receptor binding sites in developing and adult rat brain. J. Comp. Neurol. 1993;333:375–397. doi: 10.1002/cne.903330306. [ DOI ] [ PubMed ] [ Google Scholar ]
- 28. O’Kusky J., Ye P. Neurodevelopmental effects of insulin-like growth factor signaling. Front. Neuroendocrinol. 2012;33:230–251. doi: 10.1016/j.yfrne.2012.06.002. [ DOI ] [ PMC free article ] [ PubMed ] [ Google Scholar ]
- 29. Talbot K., Wang H.Y., Kazi H., Han L.Y., Bakshi K.P., Stucky A., Fuino R.L., Kawaguchi K.R., Samoyedny A.J., Wilson R.S., et al. Demonstrated brain insulin resistance in Alzheimer’s disease patients is associated with IGF-1 resistance, IRS-1 dysregulation, and cognitive decline. J. Clin. Investig. 2012;122:1316–1338. doi: 10.1172/JCI59903. [ DOI ] [ PMC free article ] [ PubMed ] [ Google Scholar ]
- 30. Bloemer J., Bhattacharya S., Amin R., Suppiramaniam V. Impaired insulin signaling and mechanisms of memory loss. Prog. Mol. Biol. Transl. Sci. 2014;121:413–449. doi: 10.1016/B978-0-12-800101-1.00013-2. [ DOI ] [ PubMed ] [ Google Scholar ]
- 31. Faria J.A., Kinote A., Ignacio-Souza L.M., de Araujo T.M., Razolli D.S., Doneda D.L., Paschoal L.B., Lellis-Santos C., Bertolini G.L., Velloso L.A., et al. Melatonin acts through MT1/MT2 receptors to activate hypothalamic Akt and suppress hepatic gluconeogenesis in rats. Am. J. Physiol. Endocrinol. Metab. 2013;305:E230–E242. doi: 10.1152/ajpendo.00094.2013. [ DOI ] [ PubMed ] [ Google Scholar ]
- 32. O’Neill C. PI3-kinase/Akt/mTOR signaling: Impaired on/off switches in aging, cognitive decline and Alzheimer’s disease. Exp. Gerontol. 2013;48:647–653. doi: 10.1016/j.exger.2013.02.025. [ DOI ] [ PubMed ] [ Google Scholar ]
- 33. Kullmann S., Heni M., Veit R., Scheffler K., Machann J., Haring H.U., Fritsche A., Preissl H. Selective insulin resistance in homeostatic and cognitive control brain areas in overweight and obese adults. Diabetes Care. 2015;38:1044–1050. doi: 10.2337/dc14-2319. [ DOI ] [ PubMed ] [ Google Scholar ]
- 34. Zhu X., Perry G., Smith M.A. Insulin signaling, diabetes mellitus and risk of Alzheimer disease. J. Alzheimer's Dis. 2005;7:81–84. doi: 10.3233/JAD-2005-7108. [ DOI ] [ PubMed ] [ Google Scholar ]
- 35. Stanley S., Wynne K., McGowan B., Bloom S. Hormonal regulation of food intake. Physiol. Rev. 2005;85:1131–1158. doi: 10.1152/physrev.00015.2004. [ DOI ] [ PubMed ] [ Google Scholar ]
- 36. Baggio L.L., Drucker D.J. Biology of incretins: GLP-1 and GIP. Gastroenterology. 2007;132:2131–2157. doi: 10.1053/j.gastro.2007.03.054. [ DOI ] [ PubMed ] [ Google Scholar ]
- 37. Varndell I.M., Bishop A.E., Sikri K.L., Uttenthal L.O., Bloom S.R., Polak J.M. Localization of glucagon-like peptide (GLP) immunoreactants in human gut and pancreas using light and electron microscopic immunocytochemistry. J. Histochem. Cytochem. 1985;33:1080–1086. doi: 10.1177/33.10.3900195. [ DOI ] [ PubMed ] [ Google Scholar ]
- 38. Holst J.J., Burcelin R., Nathanson E. Neuroprotective properties of GLP-1: Theoretical and practical applications. Curr. Med. Res. Opin. 2011;27:547–558. doi: 10.1185/03007995.2010.549466. [ DOI ] [ PubMed ] [ Google Scholar ]
- 39. Cork S.C., Richards J.E., Holt M.K., Gribble F.M., Reimann F., Trapp S. Distribution and characterisation of Glucagon-like peptide-1 receptor expressing cells in the mouse brain. Mol. Metab. 2015;4:718–731. doi: 10.1016/j.molmet.2015.07.008. [ DOI ] [ PMC free article ] [ PubMed ] [ Google Scholar ]
- 40. Abbas T., Faivre E., Holscher C. Impairment of synaptic plasticity and memory formation in GLP-1 receptor KO mice: Interaction between type 2 diabetes and Alzheimer’s disease. Behav. Brain Res. 2009;205:265–271. doi: 10.1016/j.bbr.2009.06.035. [ DOI ] [ PubMed ] [ Google Scholar ]
- 41. Hamilton A., Patterson S., Porter D., Gault V.A., Holscher C. Novel GLP-1 mimetics developed to treat type 2 diabetes promote progenitor cell proliferation in the brain. J. Neurosci. Res. 2011;89:481–489. doi: 10.1002/jnr.22565. [ DOI ] [ PubMed ] [ Google Scholar ]
- 42. McClean P.L., Parthsarathy V., Faivre E., Holscher C. The diabetes drug liraglutide prevents degenerative processes in a mouse model of Alzheimer’s disease. J. Neurosci. 2011;31:6587–6594. doi: 10.1523/JNEUROSCI.0529-11.2011. [ DOI ] [ PMC free article ] [ PubMed ] [ Google Scholar ]
- 43. Hunter K., Holscher C. Drugs developed to treat diabetes, liraglutide and lixisenatide, cross the blood brain barrier and enhance neurogenesis. BMC Neurosci. 2012;13:33. doi: 10.1186/1471-2202-13-33. [ DOI ] [ PMC free article ] [ PubMed ] [ Google Scholar ]
- 44. Hayes M.R., De Jonghe B.C., Kanoski S.E. Role of the glucagon-like-peptide-1 receptor in the control of energy balance. Physiol. Behav. 2010;100:503–510. doi: 10.1016/j.physbeh.2010.02.029. [ DOI ] [ PMC free article ] [ PubMed ] [ Google Scholar ]
- 45. Williams D.L., Baskin D.G., Schwartz M.W. Leptin regulation of the anorexic response to glucagon-like peptide-1 receptor stimulation. Diabetes. 2006;55:3387–3393. doi: 10.2337/db06-0558. [ DOI ] [ PubMed ] [ Google Scholar ]
- 46. Larsen P.J., Tang-Christensen M., Holst J.J., Orskov C. Distribution of glucagon-like peptide-1 and other preproglucagon-derived peptides in the rat hypothalamus and brainstem. Neuroscience. 1997;77:257–270. doi: 10.1016/S0306-4522(96)00434-4. [ DOI ] [ PubMed ] [ Google Scholar ]
- 47. Vrang N., Larsen P.J. Preproglucagon derived peptides GLP-1, GLP-2 and oxyntomodulin in the CNS: Role of peripherally secreted and centrally produced peptides. Prog. Neurobiol. 2010;92:442–462. doi: 10.1016/j.pneurobio.2010.07.003. [ DOI ] [ PubMed ] [ Google Scholar ]
- 48. Llewellyn-Smith I.J., Reimann F., Gribble F.M., Trapp S. Preproglucagon neurons project widely to autonomic control areas in the mouse brain. Neuroscience. 2011;180:111–121. doi: 10.1016/j.neuroscience.2011.02.023. [ DOI ] [ PMC free article ] [ PubMed ] [ Google Scholar ]
- 49. Lovshin J.A., Drucker D.J. Incretin-based therapies for type 2 diabetes mellitus. Nat. Rev. Endocrinol. 2009;5:262–269. doi: 10.1038/nrendo.2009.48. [ DOI ] [ PubMed ] [ Google Scholar ]
- 50. Vella A., Shah P., Reed A.S., Adkins A.S., Basu R., Rizza R.A. Lack of effect of exendin-4 and glucagon-like peptide-1-(7,36)-amide on insulin action in non-diabetic humans. Diabetologia. 2002;45:1410–1415. doi: 10.1007/s00125-002-0924-4. [ DOI ] [ PubMed ] [ Google Scholar ]
- 51. Oka J.I., Goto N., Kameyama T. Glucagon-like peptide-1 modulates neuronal activity in the rat’s hippocampus. Neuroreport. 1999;10:1643–1646. doi: 10.1097/00001756-199906030-00004. [ DOI ] [ PubMed ] [ Google Scholar ]
- 52. Isacson R., Nielsen E., Dannaeus K., Bertilsson G., Patrone C., Zachrisson O., Wikstrom L. The glucagon-like peptide 1 receptor agonist exendin-4 improves reference memory performance and decreases immobility in the forced swim test. Eur. J. Pharm. 2011;650:249–255. doi: 10.1016/j.ejphar.2010.10.008. [ DOI ] [ PubMed ] [ Google Scholar ]
- 53. McClean P.L., Holscher C. Liraglutide can reverse memory impairment, synaptic loss and reduce plaque load in aged APP/PS1 mice, a model of Alzheimer’s disease. Neuropharmacology. 2014;76:57–67. doi: 10.1016/j.neuropharm.2013.08.005. [ DOI ] [ PubMed ] [ Google Scholar ]
- 54. Hayes M.R. Neuronal and intracellular signaling pathways mediating GLP-1 energy balance and glycemic effects. Physiol. Behav. 2012;106:413–416. doi: 10.1016/j.physbeh.2012.02.017. [ DOI ] [ PMC free article ] [ PubMed ] [ Google Scholar ]
- 55. Salcedo I., Tweedie D., Li Y., Greig N.H. Neuroprotective and neurotrophic actions of glucagon-like peptide-1: An emerging opportunity to treat neurodegenerative and cerebrovascular disorders. Br. J. Pharm. 2012;166:1586–1599. doi: 10.1111/j.1476-5381.2012.01971.x. [ DOI ] [ PMC free article ] [ PubMed ] [ Google Scholar ]
- 56. Parthsarathy V., Holscher C. Chronic treatment with the GLP1 analogue liraglutide increases cell proliferation and differentiation into neurons in an AD mouse model. PLoS ONE. 2013;8:e58784. doi: 10.1371/journal.pone.0058784. [ DOI ] [ PMC free article ] [ PubMed ] [ Google Scholar ]
- 57. Craft S., Baker L.D., Montine T.J., Minoshima S., Watson G.S., Claxton A., Arbuckle M., Callaghan M., Tsai E., Plymate S.R., et al. Intranasal insulin therapy for Alzheimer disease and amnestic mild cognitive impairment: A pilot clinical trial. Arch. Neurol. 2012;69:29–38. doi: 10.1001/archneurol.2011.233. [ DOI ] [ PMC free article ] [ PubMed ] [ Google Scholar ]
- 58. McClean P.L., Gault V.A., Harriott P., Holscher C. Glucagon-like peptide-1 analogues enhance synaptic plasticity in the brain: A link between diabetes and Alzheimer’s disease. Eur. J. Pharm. 2010;630:158–162. doi: 10.1016/j.ejphar.2009.12.023. [ DOI ] [ PubMed ] [ Google Scholar ]
- 59. Darsalia V., Hua S., Larsson M., Mallard C., Nathanson D., Nystrom T., Sjoholm A., Johansson M.E., Patrone C. Exendin-4 reduces ischemic brain injury in normal and aged type 2 diabetic mice and promotes microglial M2 polarization. PLoS ONE. 2014;9:e103114. doi: 10.1371/journal.pone.0103114. [ DOI ] [ PMC free article ] [ PubMed ] [ Google Scholar ]
- 60. Adamska E., Ostrowska L., Gorska M., Kretowski A. The role of gastrointestinal hormones in the pathogenesis of obesity and type 2 diabetes. Przeglad Gastroenterol. 2014;9:69–76. doi: 10.5114/pg.2014.42498. [ DOI ] [ PMC free article ] [ PubMed ] [ Google Scholar ]
- 61. Ravassa S., Beaumont J., Huerta A., Barba J., Coma-Canella I., Gonzalez A., Lopez B., Diez J. Association of low GLP-1 with oxidative stress is related to cardiac disease and outcome in patients with type 2 diabetes mellitus: A pilot study. Free Radic. Biol. Med. 2015;81:1–12. doi: 10.1016/j.freeradbiomed.2015.01.002. [ DOI ] [ PubMed ] [ Google Scholar ]
- 62. Toft-Nielsen M.B., Damholt M.B., Madsbad S., Hilsted L.M., Hughes T.E., Michelsen B.K., Holst J.J. Determinants of the impaired secretion of glucagon-like peptide-1 in type 2 diabetic patients. J. Clin. Endocrinol. Metab. 2001;86:3717–3723. doi: 10.1210/jcem.86.8.7750. [ DOI ] [ PubMed ] [ Google Scholar ]
- 63. Vilsboll T., Krarup T., Deacon C.F., Madsbad S., Holst J.J. Reduced postprandial concentrations of intact biologically active glucagon-like peptide 1 in type 2 diabetic patients. Diabetes. 2001;50:609–613. doi: 10.2337/diabetes.50.3.609. [ DOI ] [ PubMed ] [ Google Scholar ]
- 64. Candeias E., Sebastiao I., Cardoso S., Carvalho C., Santos M.S., Oliveira C.R., Moreira P.I., Duarte A.I. Brain GLP-1/IGF-1 Signaling and Autophagy Mediate Exendin-4 Protection Against Apoptosis in Type 2 Diabetic Rats. Mol. Neurobiol. 2017 doi: 10.1007/s12035-017-0622-3. [ DOI ] [ PubMed ] [ Google Scholar ]
- 65. Emsley J.G., Mitchell B.D., Kempermann G., Macklis J.D. Adult neurogenesis and repair of the adult CNS with neural progenitors, precursors, and stem cells. Prog. Neurobiol. 2005;75:321–341. doi: 10.1016/j.pneurobio.2005.04.002. [ DOI ] [ PubMed ] [ Google Scholar ]
- 66. Hamilton A., Holscher C. The effect of ageing on neurogenesis and oxidative stress in the APP(swe)/PS1(deltaE9) mouse model of Alzheimer’s disease. Brain Res. 2012;1449:83–93. doi: 10.1016/j.brainres.2012.02.015. [ DOI ] [ PubMed ] [ Google Scholar ]
- 67. Cameron H.A., McKay R.D. Adult neurogenesis produces a large pool of new granule cells in the dentate gyrus. J. Comp. Neurol. 2001;435:406–417. doi: 10.1002/cne.1040. [ DOI ] [ PubMed ] [ Google Scholar ]
- 68. Abrous D.N., Koehl M., Le Moal M. Adult neurogenesis: From precursors to network and physiology. Physiol. Rev. 2005;85:523–569. doi: 10.1152/physrev.00055.2003. [ DOI ] [ PubMed ] [ Google Scholar ]
- 69. Bruel-Jungerman E., Davis S., Rampon C., Laroche S. Long-term potentiation enhances neurogenesis in the adult dentate gyrus. J. Neurosci. 2006;26:5888–5893. doi: 10.1523/JNEUROSCI.0782-06.2006. [ DOI ] [ PMC free article ] [ PubMed ] [ Google Scholar ]
- 70. Van Praag H., Shubert T., Zhao C., Gage F.H. Exercise enhances learning and hippocampal neurogenesis in aged mice. J. Neurosci. 2005;25:8680–8685. doi: 10.1523/JNEUROSCI.1731-05.2005. [ DOI ] [ PMC free article ] [ PubMed ] [ Google Scholar ]
- 71. Harkavyi A., Abuirmeileh A., Lever R., Kingsbury A.E., Biggs C.S., Whitton P.S. Glucagon-like peptide 1 receptor stimulation reverses key deficits in distinct rodent models of Parkinson’s disease. J. Neuroinflamm. 2008;5:19. doi: 10.1186/1742-2094-5-19. [ DOI ] [ PMC free article ] [ PubMed ] [ Google Scholar ]
- 72. Faure A., Verret L., Bozon B., El Tayara N.E.T., Ly M., Kober F., Dhenain M., Rampon C., Delatour B. Impaired neurogenesis, neuronal loss, and brain functional deficits in the APPxPS1-Ki mouse model of Alzheimer’s disease. Neurobiol. Aging. 2011;32:407–418. doi: 10.1016/j.neurobiolaging.2009.03.009. [ DOI ] [ PubMed ] [ Google Scholar ]
- 73. Bertilsson G., Patrone C., Zachrisson O., Andersson A., Dannaeus K., Heidrich J., Kortesmaa J., Mercer A., Nielsen E., Ronnholm H., et al. Peptide hormone exendin-4 stimulates subventricular zone neurogenesis in the adult rodent brain and induces recovery in an animal model of Parkinson’s disease. J. Neurosci. Res. 2008;86:326–338. doi: 10.1002/jnr.21483. [ DOI ] [ PubMed ] [ Google Scholar ]
- 74. Drucker D.J. Glucagon-like peptides: Regulators of cell proliferation, differentiation, and apoptosis. Mol. Endocrinol. 2003;17:161–171. doi: 10.1210/me.2002-0306. [ DOI ] [ PubMed ] [ Google Scholar ]
- 75. During M.J., Cao L., Zuzga D.S., Francis J.S., Fitzsimons H.L., Jiao X., Bland R.J., Klugmann M., Banks W.A., Drucker D.J., et al. Glucagon-like peptide-1 receptor is involved in learning and neuroprotection. Nat. Med. 2003;9:1173–1179. doi: 10.1038/nm919. [ DOI ] [ PubMed ] [ Google Scholar ]
- 76. Raber J., Rola R., LeFevour A., Morhardt D., Curley J., Mizumatsu S., VandenBerg S.R., Fike J.R. Radiation-induced cognitive impairments are associated with changes in indicators of hippocampal neurogenesis. Radiat. Res. 2004;162:39–47. doi: 10.1667/RR3206. [ DOI ] [ PubMed ] [ Google Scholar ]
- 77. Snyder J.S., Hong N.S., McDonald R.J., Wojtowicz J.M. A role for adult neurogenesis in spatial long-term memory. Neuroscience. 2005;130:843–852. doi: 10.1016/j.neuroscience.2004.10.009. [ DOI ] [ PubMed ] [ Google Scholar ]
- View on publisher site
- PDF (180.5 KB)
- Collections
Similar articles
Cited by other articles, links to ncbi databases.
- Download .nbib .nbib
- Format: AMA APA MLA NLM
Add to Collections
Type 3 Diabetes and Its Role Implications in Alzheimer's Disease
Affiliations.
- 1 Faculty of Pharmacy, Ho Chi Minh City University of Technology (HUTECH), Ho Chi Minh City 700000, Vietnam.
- 2 Institute of Research and Development, Duy Tan University, Danang 550000, Vietnam.
- 3 Faculty of Food Science and Technology, Ho Chi Minh City University of Food Industry, Ho Chi Minh City 700000, Vietnam.
- 4 Faculty of Environmental and Food Engineering, Nguyen Tat Thanh University, Ho Chi Minh City 700000, Vietnam.
- 5 Graduate School of Environment Department of Industrial and Environmental Engineering, Gachon University, 1342 Sungnam-daero, Sujung-gu, Seongnam-si, Gyeonggi-do 461-701, Korea.
- 6 Department of BionanoTechnology, Gachon Medical Research Institute, Gachon University, 1342 Sungnam-daero, Sujung-gu, Seongnam-si, Gyeonggi-do 461-701, Korea.
- PMID: 32365816
- PMCID: PMC7246646
- DOI: 10.3390/ijms21093165
The exact connection between Alzheimer's disease (AD) and type 2 diabetes is still in debate. However, poorly controlled blood sugar may increase the risk of developing Alzheimer's. This relationship is so strong that some have called Alzheimer's "diabetes of the brain" or "type 3 diabetes (T3D)". Given more recent studies continue to indicate evidence linking T3D with AD, this review aims to demonstrate the relationship between T3D and AD based on the fact that both the processing of amyloid-β (Aβ) precursor protein toxicity and the clearance of Aβ are attributed to impaired insulin signaling, and that insulin resistance mediates the dysregulation of bioenergetics and progress to AD. Furthermore, insulin-related therapeutic strategies are suggested to succeed in the development of therapies for AD by slowing down their progressive nature or even halting their future complications.
Keywords: Alzheimer’s disease; hypometabolism; insulin resistance; type 2 diabetes; type 3 diabetes.
Publication types
- Alzheimer Disease / etiology*
- Alzheimer Disease / metabolism*
- Amyloid beta-Peptides / metabolism
- Amyloid beta-Protein Precursor / metabolism
- Blood Glucose
- Brain / metabolism*
- Brain / pathology
- Diabetes Complications / metabolism*
- Diabetes Mellitus / metabolism*
- Disease Susceptibility*
- Glucagon / metabolism
- Glucose / metabolism
- Insulin / metabolism
- Insulin Resistance
- Signal Transduction
- Amyloid beta-Peptides
- Amyloid beta-Protein Precursor
An official website of the United States government
Official websites use .gov A .gov website belongs to an official government organization in the United States.
Secure .gov websites use HTTPS A lock ( Lock Locked padlock icon ) or https:// means you've safely connected to the .gov website. Share sensitive information only on official, secure websites.
- Publications
- Account settings
- Advanced Search
- Journal List

Type 3 Diabetes Mellitus: A Link Between Alzheimer's Disease and Type 2 Diabetes Mellitus
Hira pervez, bilvesh mandalia, muhammad waqas, harmandeep kaur sra.
- Author information
- Article notes
- Copyright and License information
Hira Pervez [email protected]
Corresponding author.
Received 2020 Sep 8; Accepted 2020 Nov 25; Collection date 2020 Nov.
This is an open access article distributed under the terms of the Creative Commons Attribution License, which permits unrestricted use, distribution, and reproduction in any medium, provided the original author and source are credited.
Chronic diseases, as their name suggests, are progressive and can have overlapping features. Similar to this, Alzheimer's disease (AD) and diabetes mellitus (DM) fall into the category of chronic degenerative diseases. The global burden of these two ailments is manifold; hence, it seems important to view the pathophysiologic mechanisms of DM in the worsening of AD. Genetic as well as environmental factors are seen to play a role in the disease pathogenesis. Several genes, metabolic pathways, electrolytes, and dietary habits are seen to hasten brain atrophy. Lying behind this is the accumulation of amyloid precursor and tau - the misfolded proteins - within the brain substance. This mechanism is usually innate to AD itself, but the impact of insulin resistance, disturbing the homeostatic milieu, is seen as a powerful contributing factor aggravating the neuronal loss impairing an individual's memory. Since this neuronal loss is permanent, it may lead to complications as seen with AD. To reach a consensus, we conducted an electronic literature review search using different databases. This aided us in understanding the common aspects between AD and DM on genetic, molecular, cellular levels, as well as the impact of minerals and diet on the disease manifestation. We also found that despite exceptional work, additional efforts are needed to explore the relationship between the two entities. This will help physicians, researchers, and pharmaceuticals to frame remedies targeting the cause and avoid the progression of AD.
Keywords: dementia, alzheimer’s dementia, diabetes type 2, single nucleotide polymorphism, genetics, genes, type 3 diabetes, insulin resistance
Introduction and background
Alzheimer's disease (AD) is a chronic neurodegenerative disease leading to a progressive loss of neuronal tissue. Similar to AD, type 2 diabetes mellitus (T2DM) has a similar outlook. As the global burden of the two diseases is at a rapid incline, it deems essential to study the impact of T2DM on the progression and/or worsening of AD. Some pathophysiologic mechanisms are involved in the manifestation of the two diseases, yet an overview of the genetic complexities requires special attention [ 1 ]. Many researchers around the globe can signify many genes associated with single-nucleotide polymorphisms (SNP), various metabolic pathways, and alterations in the homeostatic milieu as a reason for the exacerbation in the brain atrophy in AD with T2DM, especially with insulin resistance [ 2 ]. The scope of our review is to look for an association on the genetic level of the two diseases.
A detailed literature search was conducted using "PubMed" and "Google Scholar" as databases. "Alzheimer's Disease", "Type 3 Diabetes Mellitus", "Diabetes Mellitus Type 2", "Genes", "Single Nucleotide Polymorphisms", "Dementia" were used as MeSH terms. All the searched and reviewed articles were principally peer-reviewed, were in English, and published between January 2010 and May 2020. The initial articles were used for reference citations to provide additional supporting evidence. All the abstracts and published literature were included, and a thorough screening was performed to exclude anything beyond the scope of the manuscript. AD due to any other cause, type 1 diabetes, dementia due to other causes, and so on were part of the exclusion criteria. Full-text articles were reviewed and screened for the authenticity of the manuscript.
AD, the most common cause of late-onset dementia, is a progressive degenerative disorder of the nervous system. It is known to be the sixth leading cause of death in the United States, affecting about 5.5 million Americans [ 1 ], with around 34 million people affected worldwide [ 2 ]. Since the disease is multifactorial, a combination of both genetic and environmental aspects increases an individual’s incidence to develop this ailment in life. A salient attribute of the disease is extensive neuronal loss due to tau and Amyloid beta (A-beta) protein accumulation [ 3 ]. However, the genetic component is known to cause early-onset familial AD, one of the rare forms. Genes involving the metabolism of A-beta polymer may spontaneously mutate, leading to the early disease manifestations. Among these, the most common ones are amyloid precursor protein (APP), presenilin1 (PSEN1), and presenilin2 (PSEN2). This leads to the accumulation of oligomers of A-beta in the memory zones of the brain, especially the hippocampus and the cortex [ 4 ]. T2DM, another chronic disease, presents either as an absence of insulin most likely due to beta-cell burnout and/or insulin resistance. This may occur due to defective signaling at the cellular level, which may contribute to pancreatic burnout and hence the loss of glycemic controls [ 5 ]. The mechanism by which T2DM may involve the body is manifold. It is known to be a risk factor for many conditions such as cardiovascular, cerebrovascular diseases, and associated neuropathies [ 1 ]. Since the brain utilizes insulin, any alteration in the normal milieu can have a significant impact on this organ. This leads to an over-activation of glycogen synthase kinase-3 (GSK-3), production and modification of tau proteins, and neurofibrillary degeneration [ 6 ]. A two-fold increase in the incidence of AD has been reported in patients with T2DM [ 7 , 8 ]. Insulin helps in the degradation, transportation, and modified deposition of A-beta out of the brain. However, as defective insulin signaling can hamper these processes, it leads to abnormal accumulation of these misfolded peptides within the brain [ 9 ]. Similar to AD, T2DM is a degenerative disease leading to cellular loss within the beta cells of the pancreas. Since there are many mechanisms by which T2DM may augment AD, our main focus is to highlight certain genetic co-relations between the two of them.
Common pathophysiology of diabetes mellitus and Alzheimer's disease
Apart from the natural course of the two diseases, aging remains a high risk factor as for many other ailments. The common pathophysiology shared by the two conditions, such as insulin resistance, inflammatory stress, and aggregation of amyloid, and yet not limited to the variable cognitive alterations make it even more necessary to understand which disease has more impact on the other. By far, insulin resistance with or without diabetes remains a significant risk factor for AD. This is also related to the receptor function, where insulin-like growth factor 1 (IGF1) resistance and insulin receptor substrate (IRS) 1 and 2 dysfunctions may be triggered by the A-beta oligomers and hormonal resistance, ultimately leading to notorious cognitive decline [ 10 ]. This raises a question of whether central insulin resistance is the culprit or peripheral resistance. Both the diseases are chronic, and justifying the impact of one disease process on the other is of main interest for researchers. Literature suggests that there are around 415 million adults with T2DM, which is expected to be 640 million by 2040. Likewise, there are around 40 million demented individuals, with the proportion increasing to 110 million by 2050 [ 7 ]. The duration for the presence of AD and the appearance of symptoms with or without co-existent T2DM may provide a clue to the pathophysiological connection between the two diseases. Owing to the progressive nature of AD, it can be divided into four different stages: a stage with undetectable pathology termed as pre-disease, a preclinical stage with pathological manifestation but without cognitive decline, a stage of pre-dementia with mild cognitive impairment, and the stage of full-blown dementia. The presence of diabetes before or during these stages can predict the role of insulin resistance as a culprit for either initiation or worsening the course of AD. It is also evident from data that peripheral insulin resistance can be a contributing factor in the development of insulin resistance in the brain, leading to a reduction in the glucose uptake and ultimately increasing A-beta levels [ 11 ]. The formation of neuritic plaques is hastened in the presence of hyperinsulinemia and hyperglycemia [ 12 ]. Another entity that is seen in the literature is that the ratio of cerebrospinal fluid to blood plasma concentrations of insulin is decreased in advanced stages of AD. This is also shown in patients who have a functional loss of the APOE-4 allele. The alterations in the mitochondrial machinery remain the main link between the two diseases [ 13 ]. As an anabolic hormone, insulin has a protective role against A-beta protein accumulation within the brain as it prevents increased oxidative stress. Hence, insulin resistance especially with T2DM may be a hidden risk factor for the progression of AD, increasing the susceptibility of the brain to A-beta proteins [ 14 ].
Structure of amyloid precursor protein
A-beta (1-42) is a soluble protein. A study conducted by Baram et al. showed that amylin (1-37) seeds onto A-beta (1-42) by oligomerization. This is the product of cross-seeding among several amyloidogenic proteins leading to the formation of amylin A-beta plaques [ 3 , 15 ]. These plaques then aggregate within the brain contributing to the etiology of AD. Since both the diseases are progressive and since A-beta protein and its aggregates are degenerative proteins that accumulate chronically, these mechanisms describe why it is necessary to explore the genetic correlation between the two diseases.
Genetic association between type 2 diabetes mellitus and Alzheimer's disease
A study by Hu et al. described in detail the approach by which a genetic link can be established between the two diseases. According to the authors, understanding the association analysis versus the causation analysis is the most important aspect to describe both the diseases. In addition to this, common paths of the two disease processes need to be evaluated. These may include DNA methylation, phenotypic variations, and gene expressions. The study concluded that around 759 genes were found related to a shared genetic locus, leading to the disease process, of which 5 genes were directly and 682 were indirectly related to T2DM and AD. As indicated in the study, the authors reviewed the causative aspect that led to the diseases. Several pathways were directly or indirectly connected to the disease process. Of note were 16 pathways that directly linked both the diseases together. One pathway that caught attention was the CREBBP, MAPK, and PI3K-AKT. The enzyme RNA polymerase is directly regulated by the cyclic adenosine monophosphate response element. This response element binds to its transcriptional factor, both of which play a role in the long term memory. However, disturbance in the insulin signaling adversely impacts the working memory in patients with T2DM. GRMD1B and RP1-111D6.3 were the two main expression genes. Six phenotypes were found directly connected to the disease pathophysiology. Methylation can lead to variable gene expressions in the POU3F2, KIF4B, and TMSL3 within the dopaminergic synapses and adenosine monophosphate-activated protein kinase (AMPK) pathways leading to the genetic connection of both the diseases [ 5 ]. AMPK is known to be an energy modulator; it can stimulate the uptake of glucose in skeletal muscles and the catabolic effect of fatty acids in the adipose tissues by insulin sensitization. However, the overactivity of AMPK can lead to brain damage - modification of tau protein and loss of axonal growth [ 16 ]. Kulas et al. in their study found APP in the pancreatic tissue specifically in the pancreatic beta cells [ 17 ]. As the neurons and the beta Langerhans cells have genotypic and phenotypic similarities, the genetic expression of either organ can impact the other. Several researchers used mouse models to verify the previous studies linking insulin resistance to rapid cognitive decline owing to the pathology of AD. Two insulin-resistant states were created in transgenic mice - high-fat diet feeding and the genetic disruption of the insulin receptor substrate - to look for APP aggregate formation. It was concluded that the diseases are not just linked genetically but that diet-inducing metabolic stress and inflammation can also cause amyloid pathology [ 18 ]. Not only causation analysis but also association analysis, especially the genetic association analyses, has proven the link between these two diseases. Since T2DM is multi-factorial, the exploration of SNPs can help understand the link between these two diseases [ 9 ]. A study conducted by Silver et al. identified genes such as AKT2, PIK3CB, IGF1R, PIK3CD, MTOR, IDE, AKT1S1, AKT1, which had a significant relation with the resistance of insulin on the brain, leading to cognitive impairment. These genes were also found to have SNPs. Related to this study Silver et al. linked genetic variations that can lead to brain atrophy due to insulin resistance [ 19 ].
Type 3 diabetes mellitus
The mechanism by which insulin promotes the entry of glucose in various cells in the body is well known. Resistance to insulin hampers this process, leading to T2DM with a hyperglycemic environment. But can the same pathological process lead to the progression of AD is less well understood. However, the term “type 3 DM” is invariably used in the insulin-resistant environment prevailing in the neuropathogenic condition of AD. It is also known that AD does not have hyperglycemia and that the brain tissue is independent of glucose entry using insulin [ 20 ].
The common role of calcium in Alzheimer's disease and diabetes mellitus
A normal homeostatic milieu can be disturbed by different mechanisms, leading to the development of prospective diseases. Similarly, both T2MD and AD have a common association with an imbalance in calcium homeostasis and disease progression. “Calcium hypothesis” has caught the eye of every researcher for two decades. The link between calcium imbalance and oxidative stress governs this well-established theory. On a molecular level in AD patients, any changes that lead to free radical production is truly the reason for a sustained elevation of calcium. This impacts metabolic processes within the mitochondria, leading to premature activation of oxygenases. It has been speculated that any alteration in calcium homeostasis can lead to oxidative stress on the brain and/or pancreas similar to any cell of the body [ 21 ]. An increase in the intracellular calcium in AD can cause abnormal activation of calcium-dependent and calcium/calmodulin-dependent protein kinases, impairing the neuronal synapses and eventually leading to neuronal loss with the formation of A-beta aggregates and hyperphosphorylation of tau protein. T2DM has a similar molecular environment in terms of intracellular calcium dysregulation, leading to an additive effect when combined with AD. Some researchers used mouse genotype models to illustrate the tau phosphorylation in a diabetic environment, and this was pertinent with the imbalance of calcium, leading to impairment in the signaling within the cellular machinery [ 21 ].
Conclusions
Insulin resistance plays a cardinal role in the progression of AD. To understand this mechanism, a thorough understanding of the disease pathophysiology is required. As discussed in this review, this includes various environmental aspects such as dietary habits and the involvement of agents at the cellular level. Common genetic factors are seen to be involved in the worsening of AD in an environment of T2DM, with insulin resistance in particular. It also leads to uncontrolled inflammatory stress on the neuronal tissue, which can precipitate the formation of amyloid and tau proteins, thus worsening AD. These facts clarify the in-depth learning of AD pathophysiology in T2DM, which can assist in the prevention, therapeutic approach, and decreasing the morbidity associated with the two diseases.
The content published in Cureus is the result of clinical experience and/or research by independent individuals or organizations. Cureus is not responsible for the scientific accuracy or reliability of data or conclusions published herein. All content published within Cureus is intended only for educational, research and reference purposes. Additionally, articles published within Cureus should not be deemed a suitable substitute for the advice of a qualified health care professional. Do not disregard or avoid professional medical advice due to content published within Cureus.
The authors have declared that no competing interests exist.
- 1. Circulating mitochondrial DNA: new indices of type 2 diabetes-related cognitive impairment in Mexican Americans. Silzer T, Barber R, Sun J, Pathak G, Johnson L, O'Bryant S, Phillips N. PLoS One. 2019;14:213527. doi: 10.1371/journal.pone.0213527. [ DOI ] [ PMC free article ] [ PubMed ] [ Google Scholar ]
- 2. The projected effect of risk factor reduction on Alzheimer’s disease prevalence. Barnes DE, Yaffe K. Lancet Neurol. 2011;10:819–828. doi: 10.1016/S1474-4422(11)70072-2. [ DOI ] [ PMC free article ] [ PubMed ] [ Google Scholar ]
- 3. Amylin-Aβ oligomers at atomic resolution using molecular dynamics simulations: a link between type 2 diabetes and Alzheimer's disease. Baram M, Atsmon-Raz Y, Ma B, Nussinov R, Miller Y. Phys Chem Chem Phys. 2016;18:2330–2338. doi: 10.1039/c5cp03338a. [ DOI ] [ PMC free article ] [ PubMed ] [ Google Scholar ]
- 4. Increased susceptibility to metabolic dysregulation in a mouse model of Alzheimer’s disease is associated with impaired hypothalamic insulin signaling and elevated BCAA levels. Ruiz HH, Chi T, Shin AC, et al. Alzheimers Dement. 2016;12:851–861. doi: 10.1016/j.jalz.2016.01.008. [ DOI ] [ PMC free article ] [ PubMed ] [ Google Scholar ]
- 5. Shared causal paths underlying Alzheimer's dementia and type 2 diabetes. Hu Z, Jiao R, Wang P, et al. Sci Rep. 2020;10:4107. doi: 10.1038/s41598-020-60682-3. [ DOI ] [ PMC free article ] [ PubMed ] [ Google Scholar ]
- 6. Common neurodegenerative pathways in obesity, diabetes, and Alzheimers disease. Pugazhenthi S, Qin L, Reddy PH. Biochim Biophys Acta Mol Basis Dis. 2017;1863:1037–1045. doi: 10.1016/j.bbadis.2016.04.017. [ DOI ] [ PMC free article ] [ PubMed ] [ Google Scholar ]
- 7. Diabetes and cognitive impairment. Zilliox LA, Chadrasekaran K, Kwan JY, Russell JW. Curr Diab Rep. 2016;16:87. doi: 10.1007/s11892-016-0775-x. [ DOI ] [ PMC free article ] [ PubMed ] [ Google Scholar ]
- 8. Diabetes mellitus and the risk of dementia: the Rotterdam study. Ott A, Stolk RP, van Harskamp F, Pols HA, Hofman A, Breteler MM. Neurology. 1999;53:1937–1942. doi: 10.1212/wnl.53.9.1937. [ DOI ] [ PubMed ] [ Google Scholar ]
- 9. Brain insulin resistance deteriorates cognition by altering the topological features of brain networks. Su F, Shu H, Ye Q, et al. https://www.ncbi.nlm.nih.gov/pmc/articles/PMC5192246/ Neuroimage Clin. 2016;12:280–287. doi: 10.1016/j.nicl.2016.12.009. [ DOI ] [ PMC free article ] [ PubMed ] [ Google Scholar ]
- 10. Demonstrated brain insulin resistance in Alzheimer's disease patients is associated with IGF-1 resistance, IRS-1 dysregulation, and cognitive decline. Talbot K, Wang HY, Kazi H, et al. J Clin Invest. 2012;162:1316–1338. doi: 10.1172/JCI59903. [ DOI ] [ PMC free article ] [ PubMed ] [ Google Scholar ]
- 11. Insulin resistance and Alzheimer-like reductions in regional cerebral glucose metabolism for cognitively normal adults with prediabetes or early type 2 diabetes. Baker LD, Cross DJ, Minoshima S, Belongia D, Watson GS, Craft S. Arch Neurol. 2011;68:51–57. doi: 10.1001/archneurol.2010.225. [ DOI ] [ PMC free article ] [ PubMed ] [ Google Scholar ]
- 12. Insulin resistance is associated with the pathology of Alzheimer disease: the Hisayama study. Matsuzaki T, Sasaki K, Tanizaki Y, et al. Neurology. 2010;75:764–770. doi: 10.1212/WNL.0b013e3181eee25f. [ DOI ] [ PubMed ] [ Google Scholar ]
- 13. Brain insulin dysregulation: implication for neurological and neuropsychiatric disorders. Ghasemi R, Dargahi L, Haeri A, Moosavi M, Mohamed Z, Ahmadiani A. Molecular Neurobiology. 2013;47:1045–1065. doi: 10.1007/s12035-013-8404-z. [ DOI ] [ PubMed ] [ Google Scholar ]
- 14. Molecular indices of oxidative stress and mitochondrial dysfunction occur early and often progress with severity of Alzheimer's disease. de la Monte SM, Wands JR. J Alzheimers Dis. 2006;9:167–181. doi: 10.3233/jad-2006-9209. [ DOI ] [ PubMed ] [ Google Scholar ]
- 15. Seeding specificity in amyloid growth induced by heterologous fibrils. O'Nuallain B, Williams AD, Westermark P, Wetzel R. J Biol Chem. 2004;279:17490–17499. doi: 10.1074/jbc.M311300200. [ DOI ] [ PubMed ] [ Google Scholar ]
- 16. AMPK in neurodegenerative diseases. Domise MV, Vingtdeux V. Exp Suppl. 2016;107:153–177. doi: 10.1007/978-3-319-43589-3_7. [ DOI ] [ PubMed ] [ Google Scholar ]
- 17. Amyloid precursor protein in pancreatic islets. Kulas JA, Puig KL, Combs CK. J Endocrinol. 2017;235:49–67. doi: 10.1530/JOE-17-0122. [ DOI ] [ PMC free article ] [ PubMed ] [ Google Scholar ]
- 18. Clusters of conserved beta cell marker genes for assessment of beta cell phenotype. Martens GA, Jiang L, Hellemans KH, et al. PLoS ONE. 2011;6:24134. doi: 10.1371/journal.pone.0024134. [ DOI ] [ PMC free article ] [ PubMed ] [ Google Scholar ]
- 19. Identification of gene pathways implicated in Alzheimer's disease using longitudinal imaging phenotypes with sparse regression. Silver M, Janousova E, Hua X, Thompson PM, Montana G, Alzheimer's Disease Neuroimaging Initiative. Neuroimage. 2012;63:1681–1694. doi: 10.1016/j.neuroimage.2012.08.002. [ DOI ] [ PMC free article ] [ PubMed ] [ Google Scholar ]
- 20. Alzheimer's disease is type 3 diabetes-evidence reviewed. de la Monte SM, Wands JR. J Diabetes Sci Technol. 2008;2:1101–1113. doi: 10.1177/193229680800200619. [ DOI ] [ PMC free article ] [ PubMed ] [ Google Scholar ]
- 21. Chronic diabetic states worsen Alzheimer neuropathology and cognitive deficits accompanying disruption of calcium signaling in leptin-deficient APP/PS1 mice. Zhang S, Chai R, Yang YY, et al. Oncotarget. 2017;8:43617–43634. doi: 10.18632/oncotarget.17116. [ DOI ] [ PMC free article ] [ PubMed ] [ Google Scholar ]
- View on publisher site
- PDF (109.9 KB)
- Collections
Similar articles
Cited by other articles, links to ncbi databases.
- Download .nbib .nbib
- Format: AMA APA MLA NLM
Add to Collections
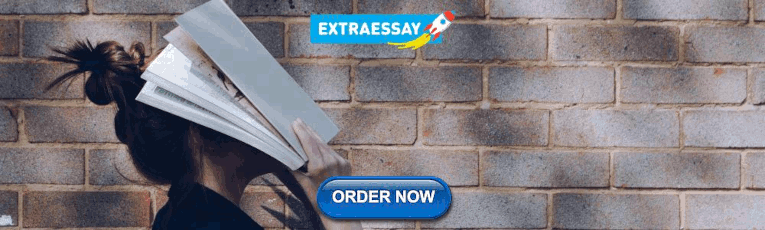
IMAGES
VIDEO
COMMENTS
Some epidemiological studies suggest that insulin resistance increases the risk for dementia and AD, even in nondiabetic populations. A recently discovered form has been suggested to be termed type 3 diabetes mellitus (T3DM) by scientists.
Researchers wondered if it is diabetes of the brain, could insulin delivered in an intranasal mist help patients? The results of a phase 2 clinical trial have raised hope. " The outcome is very positive. The patient's cognitive decline is slowed, if not improved."
Type 3 diabetes is a term used by some researchers to describe the theory that insulin resistance and insulin-like growth factor dysfunction in the brain may cause Alzheimer’s disease.
Several studies have demonstrated that GLP-1 alleviates learning and memory dysfunction by enhancing the regulation of glucose in the AD brain. However, the specific actions of GLP-1 in the AD brain are not fully understood. Here, we review evidences related to the role of GLP-1 in type 3 diabetes.
The exact connection between Alzheimer's disease (AD) and type 2 diabetes is still in debate. However, poorly controlled blood sugar may increase the risk of developing Alzheimer's. This relationship is so strong that some have called Alzheimer's "diabetes of the brain" or "type 3 diabetes (T3D)".
To reach a consensus, we conducted an electronic literature review search using different databases. This aided us in understanding the common aspects between AD and DM on genetic, molecular, cellular levels, as well as the impact of minerals and diet on the disease manifestation.