
An official website of the United States government
The .gov means it’s official. Federal government websites often end in .gov or .mil. Before sharing sensitive information, make sure you’re on a federal government site.
The site is secure. The https:// ensures that you are connecting to the official website and that any information you provide is encrypted and transmitted securely.
- Publications
- Account settings
Preview improvements coming to the PMC website in October 2024. Learn More or Try it out now .
- Advanced Search
- Journal List

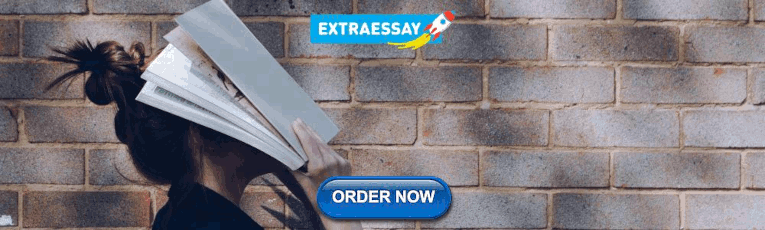
Zebrafish: A Pharmacological Model for Learning and Memory Research
Jen kit tan.
1 Department of Biochemistry, Faculty of Medicine, Universiti Kebangsaan Malaysia (UKM), UKM Medical Center, Kuala Lumpur 56000, Malaysia
Faris Hazwan Nazar
Suzana makpol, seong lin teoh.
2 Department of Anatomy, Faculty of Medicine, Universiti Kebangsaan Malaysia (UKM), UKM Medical Center, Kuala Lumpur 56000, Malaysia
Associated Data
Not applicable.
Learning and memory are essential to organism survival and are conserved across various species, especially vertebrates. Cognitive studies involving learning and memory require using appropriate model organisms to translate relevant findings to humans. Zebrafish are becoming increasingly popular as one of the animal models for neurodegenerative diseases due to their low maintenance cost, prolific nature and amenability to genetic manipulation. More importantly, zebrafish exhibit a repertoire of neurobehaviors comparable to humans. In this review, we discuss the forms of learning and memory abilities in zebrafish and the tests used to evaluate the neurobehaviors in this species. In addition, the pharmacological studies that used zebrafish as models to screen for the effects of neuroprotective and neurotoxic compounds on cognitive performance will be summarized here. Lastly, we discuss the challenges and perspectives in establishing zebrafish as a robust model for cognitive research involving learning and memory. Zebrafish are becoming an indispensable model in learning and memory research for screening neuroprotective agents against cognitive impairment.
1. Introduction
Zebrafish ( Danio rerio ) are tropical fish native to southern Asia and became a vertebrate model organism in developmental biology pioneered by George Streisinger in the 1970s at the University of Oregon. Since then, zebrafish have emerged as one of the animal models in preclinical studies for understanding various physiological processes and diseases. The growing interest is attributed to the favorable features offered by this species. These include ex vivo fertilization, transparent embryos and larvae (facilitate imaging), small size (2–5 cm for adult), easy maintenance, prolific nature (more than 100 eggs produced per fish), rapid development (larvae start to swim freely at 5 days post fertilization, dpf), their accessibility for genetic manipulations such as CRISPR and high homology (70%) to the human genome [ 1 , 2 , 3 ]. These features allow the setting up of laboratory facilities and experiments at a low cost [ 4 ]. Hence, zebrafish animal models provide an unprecedented opportunity for medium- to high-throughput screening in drug discovery for numerous disease models, such as cancer, organ regeneration and neurodegenerative disorders [ 3 , 5 , 6 ].
Dementia is the progressive deterioration of cognitive functions, including learning and memory processes, beyond the usual rate of aging. Age is the major risk factor for dementia. Alzheimer disease (AD) is the most prevalent form of dementia (60–70%) that affects more than 57 million people globally [ 7 ]. The two main classes of AD are familial AD and sporadic AD. Familial AD accounts for less than 5% of AD cases. Its main hallmarks are mutations involving amyloid precursor protein, presenilin 1 and presenilin 2. Sporadic AD is associated with environmental factors and genetic susceptibilities such as the apolipoprotein E genotype [ 8 , 9 , 10 ]. Despite the intensive efforts in drug development for AD, effective interventions remain limited. Some of the main challenges are the diverse etiology and complex risk factors in AD. Furthermore, the lack of biomarkers for early diagnosis means the disease has to progress to an advanced stage until the manifestation of signs and symptoms can be identified [ 11 ]. One of the main objectives of AD research is the development of medications that can slow or improve the main symptom of AD, i.e., memory lapses.
Zebrafish show a high degree of conservation in the neuroanatomical organization and neurotransmitter signaling pathways with humans [ 10 , 11 ]. The dorsal, medial and lateral pallium of zebrafish correspond to the isocortex, amygdala and hippocampus in mammals, respectively. The zebrafish encephalon is divided into diencephalon (forebrain), telencephalon (midbrain) and cerebellum (hindbrain). Zebrafish also retain the main excitatory glutamatergic and inhibitory GABAergic neurotransmissions and express muscarinic cholinergic receptors [ 10 ]. Neurobehaviors related to learning and memory were first reported over two decades ago [ 12 ]. Since then, a repertoire of behaviors comparable to a human, such as locomotor activity, anxiety-like behaviors, learning, memory retention, spatial and object recognition, fear responses and social preference and interaction, has been characterized in this animal [ 10 ]. These features have opened up the chance to engage zebrafish as an alternative vertebrate model in cognitive decline-related research, especially for drug discovery.
Non-human primates and rodents are the classical animal models for behavioral studies involving cognition [ 13 ]. Non-human primates have the closest neurobehavioral profiles to humans, but these studies are the costliest among other model organisms and are subject to strict ethical considerations. Rodents have been traditionally used as genetic and pharmacological models in cognitive research, but the experimental cost limits their application in large-scale drug screening. Non-mammalian organisms, such as teleost fish, fruit flies and honeybees, are being used as a complementary model because of their low costs, which are amenable to high-throughput screening [ 14 ]. Zebrafish is the most popular non-mammalian model for cognitive research because it is a vertebrate and displays a repertoire of neurobehaviors that can be related to humans. Nonetheless, findings from zebrafish studies require further validation in mammalian models before being translated into clinical trials.
This review describes the cognitive behaviors related to learning and memory in zebrafish and the assessment tools available for these behaviors. We summarize pharmacological studies that used zebrafish models to screen for the effects of neuroprotective and neurotoxic compounds on cognitive performance. Challenges and perspectives in establishing zebrafish as a robust model to study cognitive impairment are also discussed.
2. Types of Learning and Memory in Zebrafish
Cognition is the mental process of acquiring knowledge and understanding through thinking, learning, memorizing and sensing. The cognitive process includes sensation, perception, motor skills, attention, memory, executive function, language and processing speed [ 15 ]. Learning and memory performance is one of the most commonly assessed cognitive domains in AD research for drug discovery. Learning is the process of acquiring new information through the formation of memory, while memory is the process of consolidating, storing and recalling the acquired information [ 16 ]. Learning and memory are not the same, but both terms are often used interchangeably, especially in animal studies, because both processes are highly interdependent and difficult to distinguish and interpret alone. In this review, learning and memory are regarded as one integrated functional domain unless specified otherwise.
Zebrafish display different learning abilities, such as associative, non-associative, social (shoaling) and motor learning. In social learning, a group of zebrafish learned faster than a single individual [ 17 ]. In motor learning, zebrafish adapted locomotor commands to execute accurate movements that relied on sensory feedback [ 18 ]. This section will only focus on associative and non-associative learning because their paradigms (especially associative learning) are widely used to assess learning and memory performance in zebrafish pharmacological models ( Table 1 ).
Types of non-associative and associative learning.
2.1. Non-Associative Learning
Non-associative learning is a general lasting change in response strength toward a stimulus due to repeated exposure. Non-associative learning is further categorized into habituation and sensitization. Habituation is the decrease while sensitization is the increase in the animal’s response to a sensory stimulus upon continuous exposure. The changes in responses could be in the short- and long-term. Habituation has been regarded as an evolutionarily conserved behavior for optimal survival. Habituation allows the animal to disregard repeated stimuli while focusing on important stimuli such as potential predators and danger in the environment [ 1 , 19 ]. In the laboratory setting, prolonged exposure to a series of acoustic stimuli [ 19 ] and reduced light of the surrounding environment [ 26 ] reduced the startle response in zebrafish larvae. The larvae were habituated to the sensory stimuli and could exhibit non-associative learning as early as 5 to 7 dpf. In contrast, sensitization is not commonly reported in zebrafish studies. A previous study has used sensitization to assess drug addiction. Pisera-Fuester and coworkers showed that repeated administration of nicotine and cocaine at sub-threshold doses increased the zebrafish locomotor activity and sensitivity in a subsequent paradigm known as conditioned place preference (a contextual associative conditioning with appetitive stimulus) [ 20 ]. The sensitization procedure has potentiated the induction of addiction in the animal.
2.2. Associative Learning
Associative learning is the process of acquiring new information by linking two elements. This type of learning is more commonly assessed than non-associative learning in zebrafish studies. The two forms of associative learning are classical (Pavlovian) and operant (instrumental) conditioning [ 1 , 16 , 27 ]. In classical conditioning, an initially neutral stimulus is repeatedly paired with an unconditional stimulus (US) that elicits an involuntary reflex response (unconditioned response) until the neutral stimulus triggers the response upon subsequent exposure. The neutral stimulus has become the conditional stimulus (CS) that initiates the conditioned response when the animal learns to associate the CS with the US after repeated pairing.
In operant conditioning, an animal learns to correlate its voluntary behavioral responses with their consequences [ 28 ]. The term was first introduced by B.F. Skinner in 1937 to define associative learning made between a behavior and its consequence, which differed from classical conditioning [ 29 ]. The delivery of an US is determined by the behavioral response to modify (strengthen or weaken) the operant behavior. The US can be paired with a neutral stimulus until the administration of the neutral stimulus alone can trigger the conditioned response. In the end, the behavioral responses that result in favorable consequences are reinforced, whereas those that lead to undesirable outcomes are weakened.
2.2.1. Classical Conditioning
Classical conditioning can be further divided into appetitive and aversive conditioning based on the nature of the US. Appetitive conditioning utilizes favorable stimuli as the US. Sison and Gerlai demonstrated that zebrafish are capable of associative learning by using food as the reward and a red card as the visual cue [ 30 ]. After the conditioning, the zebrafish spent more time in the target arm based on the position of the red card that was previously paired with the food during the training session. Although the authors classified the learning as classical conditioning, the preference was scored based on the time spent in the targeted arm. Precisely, the response should be considered as voluntary, such that the zebrafish had to perform the behavior (choose the correct arm) to receive the outcome. To our knowledge, there is hardly any paradigm that actually evaluates the classical appetitive conditioning in zebrafish, because the involuntary responses toward rewards (food or sight of conspecific) in zebrafish have not been well-characterized so far.
In aversive conditioning, the CS is paired with a fear-inducing US that leads to a fear-related conditioned response when the CS is administered alone. The nature of the CS can be contextual (location or environment) or cued (sensory like visual cues). The most commonly used aversive US in zebrafish studies is the application of electric shock (ES). Fear conditioning is the most common form of classical learning assessed in zebrafish. Valente and colleagues demonstrated that both larval and adult zebrafish could perform cued fear conditioning in a paradigm that associated a checkerboard pattern (CS) with the ES (US) [ 31 ]. After training, the fish exhibited a conditioned fear response by preferring the non ES-paired area when the visual cue was administered alone. The classical learning started as early as 3 weeks post fertilization (wpf), and the performance reached a level comparable to 1 year-old adult fish by 6 wpf. Although the authors considered this form of learning as classical conditioning, the performance index was scored based on the animal’s position and turning behavior that should be regarded as voluntary responses.
In contextual fear conditioning, a novel environment is associated with the US. Kenney and colleagues showed that contextual fear conditioning in zebrafish lasted for at least 14 days and varied in the fear extinction rates among different strains [ 21 ]. Extinction is a process in associative learning such that repeated exposure to the CS without the US will eventually reduce the conditioned response. The fear response was evaluated based on the distance traveled in the tank (locomotion activity) which can be considered as an involuntary behavior. Classical fear conditioning of TU zebrafish strain extinguished more quickly than AB and TL background strains.
Fear-related responses include freezing (immobility), erratic movements (zigzagging), bottom-dwelling, a tighter shoal, leaping (jumping) and thigmotaxis [ 32 ]. In the study by Baker and Wong, they used the alarm substance released from the fish skin when injured as an olfactory cue (US) to trigger anti-predatory (fear) responses such as freezing duration and erratic movements [ 22 ]. Interestingly, contextual fear learning and memory in zebrafish differed by the stress coping styles. Fear memory was acquired faster in zebrafish with a reactive stress coping style than in proactive fish. The authors postulated that a faster learning rate in reactive fish might facilitate memory encoding. The reactive fish are more sensitive to environmental cues by perceiving the fear stimulus as more threatening and becoming more risk-averse. This may lead to longer fear memory retention in the reactive fish as observed in the study. At the molecular level, the influence of stress coping styles on learning and memory performance could be explained by the difference in the transcriptomic state of the zebrafish brain. The reactive fish brain showed increased expression of genes related to synaptic plasticity and neurotransmission [ 33 ]. Maximino et al. reported that neurotransmitters such as epinephrine and norepinephrine in the autonomic nervous system and extracellular serotonin levels in the zebrafish brain increased after the exposure of conspecific alarm substance [ 34 ]. The study demonstrated that fear-induced responses and sympathetic activation were mediated by the serotonin transporter.
2.2.2. Operant Conditioning
Operant conditioning can be classified as reinforcement or punishment, such that the paradigm increases or decreases the response to modify the strength of the response over the course of training. The reinforcement in operant conditioning can be positive (addition of reward as a reinforcer) or negative (removal of aversive stimulus as a reinforcer). Both positive and negative reinforcement paradigms are aimed at strengthening the behavioral response.
Positive reinforcement administers the appetitive US after the animal performs a behavioral response to strengthen the response. Manabe and co-workers developed an automated operant device for positive reinforcement conditioning in zebrafish. The response key was equipped with a sensor that dispenses brine shrimp eggs when it was approached by zebrafish [ 23 ]. In the paradigm, the red LED attached to the response key was first illuminated and then switched off when the fish approached the response key, followed by the dispensation of food.
Negative reinforcement removes the aversive US when the animal engages in a behavioral response. This type of conditioning can be further divided into escape learning and avoidance (active and passive). Escape learning refers to the animal engages a behavior to terminate the ongoing aversive stimulus. For example, the crossing response of rodents will be increased from a compartment with existing foot shock to the opposite compartment in a shuttle box. The active avoidance paradigm requires the presentation of a cue (visual/auditory/contextual) prior to the administration of an aversive stimulus. Active avoidance was performed to prevent the happening of the aversive stimulus. Xu and colleagues demonstrated that zebrafish increased crossing response to a compartment without ES upon presenting a light signal previously paired with the administration of ES [ 24 ]. In passive avoidance, also known as inhibitory avoidance, the conditioning suppresses an innate behavior to avoid the occurrence of an aversive stimulus. For example, zebrafish have an innate response to enter a dark environment (scototaxis) [ 25 ]. In the paradigm, the ES was applied when the fish entered the dark compartment of a tank. After the conditioning, the latency to enter the dark compartment was increased [ 25 , 35 ].
Similarly, punishment-based operant conditioning is divided into positive (impose punishment) and negative (remove appetitive stimulus as punishment). Both positive and negative punishment paradigms are aimed at weakening the behavioral response. For positive punishment, an aversive US is administered when a behavioral response is engaged, eventually leading to a decreased behavioral response. The difference between positive punishment, escape learning and avoidance is that the behavior response in positive punishment leads to the administration of an aversive stimulus, while the behavioral response in the latter two negative reinforcement results in the removal/prevention of an aversive stimulus. For example, in positive punishment conditioning, a rodent learns to refrain from pressing a lever that will lead to foot shock.
For negative punishment, an appetitive US is removed when the animal performs a behavioral response. For instance, this conditioning is used in dog training to correct undesired behavior by taking away the reward (food or toy). To our knowledge, both positive and negative punishments have not been engaged in pharmacological studies using zebrafish as models to assess cognitive performance.
2.3. Remarks on Leaning Conditionings in Zebrafish
In summary, zebrafish are capable of performing both non-associative and associative learning tasks. This allows the researcher to assess the zebrafish’s cognitive performance using various tests. From a survival perspective, operant learning allows the animals to find a safe and rewarding outcome while avoiding danger in a complex environment. Unsurprisingly, operant conditioning is pervasive in zebrafish studies because task performance could be enhanced by adding an operant component [ 31 ]. Nevertheless, not all types of operant conditioning can be assessed in zebrafish as in the rodent models due to lack of a suitable operant box that can be accessed by many laboratories, In addition, the nature (involuntary and voluntary) of some behaviors, especially for classical conditioning, in zebrafish is not clearly defined (for example, location preference, time spent). In a review by Pritchett and Brenna, the authors considered that many behavioral tasks in zebrafish are actually a hybrid of classical and operant conditioning [ 27 ]. In this review, tasks that include both involuntary and voluntary behaviors, and assess these responses from a hybrid of classical and operant conditioning are simply referred as associative learning. Precise classification of associative learning might be important when understanding the distinct neural circuits involved. Future studies are encouraged to indicate the types of associative learning if possible.
2.4. Memory
Memory can be classified mainly into sensory, short-term and long-term [ 36 , 37 ]. Sensory memory is further divided into haptic (based on sight stimuli), echoic (based on auditory stimuli) and iconic (based on touch stimuli) memory. Based on the duration of holding, memory is divided into short- and long-term. Working memory is a form of short-term memory that not only temporarily retains but manipulates the information for cognition, including learning, reasoning and language comprehension. Long-term memory can be further grouped as declarative (explicit or conscious) and non-declarative (implicit or unconscious) memory. There are two types of declarative memory, namely episodic (stores personal experience) and semantic (stores facts and conceptual knowledge) memory. The four types of non-declarative memory include procedural (recalling motor and executive skills), associative, non-associative and priming (influence of a pre-exposed/suggested stimulus on response to the next stimulus) memory.
Not all forms of memory can be evaluated in zebrafish as model organisms. Similar to rodent models, the most common types of memory assessed in zebrafish for cognitive studies are spatial, recognition and associative memory. To score the memory retention index, a time delay between the training (familiarization) and test (probe) phases is required. Spatial memory in zebrafish is assessed based on the preference of zebrafish for new space/area. The cue to a novel place could be contextual or visual (such as geometric cues of circle, triangle and square around the tank). Cognato et al. showed that spatial memory in zebrafish lasted up to 3 h after the familiarization session and diminished after 6 h of exposure [ 38 ]. Objective recognition memory in zebrafish refers to the preference of zebrafish for a new object. By using virtual objects presented via iPod, Braida et al. demonstrated that object recognition memory lasted up to 24 h post-familiarization session [ 39 ]. Interestingly, Madeira and Oliveira reported that zebrafish were capable of social recognition memory, i.e., the preference for new conspecifics, in a social discrimination paradigm [ 40 ]. The zebrafish were exposed to two conspecifics via perforated partitions such that the recognition relied on visual and olfactory cues. Zebrafish had increased exploration time toward novel conspecifics than familiar conspecifics after 24 h post-familiarization session. Associative memory in zebrafish based on passive avoidance [ 25 ] and appetitive conditioning [ 41 ] has been reported. Previous studies also explored other more complex forms of memory such as working memory [ 42 , 43 ] and episodic-like memory [ 44 ] in zebrafish. As these forms of memory are challenging to be defined and tested in animals, more evidence is required to confirm the presence of these forms of memory in zebrafish.
3. Behavioral Assessment Tests in Zebrafish
The main types of behaviors assessed in zebrafish as pharmacological models for learning and memory performance are locomotion, emotion (anxiety-like), cognition (learning, object recognition, spatial memory) and social interaction (preference, aggressiveness) [ 45 ]. ( Table 2 ) There could be some variations when conducting a neurobehavioral paradigm in zebrafish to capture the change of innate behavioral response due to the drug treatment. For example, the habituation (familiarization) phase might be employed in a conditioning paradigm to reduce the anxiety or exploration of zebrafish toward novel environments. However, not all studies engaged a habituation phase, or the habituation duration might be varied between studies. A training phase is employed for memory acquisition and consolidation. In associative conditioning paradigms, the learning performance can be assessed throughout training if the paradigms involve multiple training sessions. For example, the effect of a drug on the behavioral response can be compared based on the difference between pre- and post-training [ 46 ]. Then, memory retention is evaluated in a probe phase which is conducted after the last training session. The time interval between the training and probe phase can be adjusted to evaluate short- and long-term memory. The probe phase is conducted without the unconditioned stimulus, while the conditioned response is recorded as an indicator of memory retention based on the difference between treated and untreated groups [ 47 ].
Neurobehavioral assessment tests in zebrafish.
3.1. Locomotor Activity Test
The locomotor activity test (LAT) is used to evaluate the spontaneous swimming behaviors of zebrafish [ 48 ]. It is not a learning and memory test but is often conducted in line with cognitive tests because locomotor activity is involved in cognitive tests in response to stimuli. In addition, psychoactive drugs often impact locomotor activity. LAT is used to establish the baseline locomotor activity, as well as the effect of the drugs and environmental stimuli on locomotor activity, which may impact the cognitive test results later [ 49 ]. LAT is conducted by holding a single zebrafish in a trapezoid or rectangle water tank for a few minutes. The movement of zebrafish is recorded from the front (usually) or top of the tank, and the data are analyzed using tracking software. Parameters assessed in the LAT include total distance traveled, swimming speed (m/s, average or maximum), freezing time and thigmotaxis (time spent and distance traveled near the tank wall). A 3D LAT was developed to track the spatial movement of zebrafish [ 50 ].
LAT is generally performed first in a battery of behavioral tests before other more complicated paradigms. This can prevent the animals from becoming exhausted and less motivated to perform the subsequent paradigms. To our knowledge, there is no consensus on a standardized environment or environmental conditions for LAT. Most behavioral tests developed for zebrafish serve as a general guide rather than a standardized protocol. Considering that zebrafish are increasingly used as study models across different laboratories worldwide, standardization may be necessary to make the findings from different studies comparable. Recently, Maeda et al. developed a standardized method for assessing the behavioral response (including locomotor activity/response) in zebrafish larvae (6 to 7 dpf) using a light–dark locomotion test [ 49 ]. The characterized responses were proposed to be used for screening psychoactive substances. In addition, Ogi et al. suggested some recommendations/guidelines for conducting a social preference test based on their systematic review [ 51 ]. Nevertheless, we think it is challenging to follow exactly a standardized protocol such that certain modifications might be required. The adaptation could be attributed to the strain/source of zebrafish, the pharmacokinetics of drugs being tested (hence the timing to evaluate the drug efficacy concerning the paradigm) and the test apparatus and setup (water exchange frequency and temperature; the same types/setup of stimuli being not available). Therefore, it is recommended to specify these parameters as detailed as possible in the publication to improve the reproducibility of the test results. More importantly, the inclusion of positive and negative drug control groups is highly advisable.
3.2. Novel Tank Test
A novel tank test is, similar to the LAT setup, used to assess the anxiety-like behavior of zebrafish [ 48 ]. The tank is divided horizontally into two segments during the data analysis. Zebrafish tend to stay in the lower segment of the tank at the beginning of the test (geotaxis) and then start to explore the upper part. The reduction of time spent and distance traveled in the upper half of the tank is an indication of the anxiety-like behavior in zebrafish. Anxiogenic drugs that severely affect zebrafish exploration could impact the fish’s motivation to perform a cognitive test.
3.3. Inhibitory Avoidance Test
The inhibitory avoidance test (IAT) is one of the most commonly used cognitive tests to evaluate the learning and memory functions of zebrafish. The test can be established using a two-chamber tank ( Figure 1 A) and a maze tank of various shapes ( Figure 1 B–D). In a two-chamber tank, both sides of the tank/maze are cued with color sleeves or a dark–light environment, while one side is chosen to pair the cue with an aversive stimulus such as the ES [ 52 ], dropping weight [ 53 ] or stirring [ 54 ]. In IAT, the favorable side (usually the dark compartment) is chosen for the CS–US pairing. During the training session, the zebrafish is placed in the light compartment and allowed to cross over to the dark compartment. An ES is applied to fish when it enters the dark compartment. The training session can be repeated several times until a conditioned response develops. A probe session is then conducted after 24 h (for short-term memory) of the last training trial, such that the fish is allowed to explore the compartment without the ES. The latency to enter the dark compartment during the training and probe sessions is recorded as an indication of memory retention. Other parameters such as the number of entries to and distance traveled in the dark compartment can be measured using software.

Types of tank or maze used for cognitive tests in zebrafish. ( A ) Two-chamber tank colored coded as a visual cue, for example. The unconditioned stimulus can be appetitive such as a food reward, or aversive such as an electric shock; ( B ) T-maze; ( C ) Plus-maze; ( D ) Y-maze. The arms of the mazes can be coded with different color or geometric cues.
3.4. Appetitive Conditioning Test
Similar to IAT, a rectangle tank or maze is used to establish the CS–US pairing over repeated exposure. Chen et al. covered the left and right arms of a T-maze with green and red colored sleeves as visual cues [ 48 ]. One of the arms was used as an enriched chamber that contained brine shrimp as the appetitive stimulus. The test paradigm consisted of habituation, training and probe phases. The authors evaluated the memory performance based on the latency to enter and time spent in the targeted zone. Other appetitive stimuli can be the sight of conspecifics, favorable environment with artificial grass and stones.
3.5. Y-Maze Test
The Y-maze test is used to inspect the spatial memory of zebrafish based on their innate behavior that tends to explore the novel place [ 55 ]. Zebrafish were tested individually in a Y-shaped tank. Spatial memory was represented by spontaneous alternation percentage (tendency to alternate different arms in every three consecutive entries), time spent and distance traveled in the novel arm.
3.6. Novel Object Recognition Test
Similar to the Y-maze that is based on the fish response to novelty, a novel object recognition test is used to examine the recognition memory. In the test conducted by Capatina et al., a zebrafish was placed in a tank with two familiar objects (red cubes) and allowed to explore the tank for 10 min [ 55 ]. After an hour, one of the red cubes (familiar objects) was replaced by a green cube (novel object) and the exploration of fish was recorded. Distance less than 2.5 cm away from the objects was considered as an exploration of the objects. Recognition memory was scored by the exploratory time and the preference for the novel object.
4. Zebrafish Models for Pharmacological Studies on Learning and Memory
4.1. neuroprotective screening.
The neurobehavioral tests for zebrafish have been reported for over two decades [ 12 ]. The utilization of zebrafish as a model to screen for compounds with a neuroprotective effect against cognitive impairment started a decade ago [ 53 ]. In recent years, zebrafish have become one of the popular animal models for screening the effects of neuroprotective drugs against various cognitive impairment models [ 47 , 53 , 54 , 55 , 56 , 57 , 58 , 59 , 60 , 61 , 62 , 63 , 64 , 65 , 66 , 67 , 68 , 69 , 70 , 71 , 72 , 73 , 74 , 75 , 76 , 77 , 78 , 79 ]. ( Supplementary Table S1 ) This is attributed to the development of robust behavioral tests and well-characterized neurobehaviors and signaling pathways in zebrafish. Different zebrafish models have been developed to represent cognitive impairment in humans. These models are useful in screening for agents with neuroprotective effects that restore learning and memory performance.
Cholinergic signaling is involved in memory acquisition and consolidation. Reduction of acetylcholine (ACh) due to hydrolysis by acetylcholinesterase (AChE) could implicate the development of dementia and AD. Scopolamine is an alkaloid that acts as a nonselective muscarinic receptor antagonist. It blocks the receptors to induce cognitive impairment. Scopolamine-induced amnesia in zebrafish is a widely used model for screening drugs with neuroprotective effects [ 53 ]. Capatina et al. investigated the effects of oregano ( Origanum vulgare spp. hirtum (Lamiaceae)) essential oil on the scopolamine-induced amnesia zebrafish model [ 55 ]. Scopolamine fish pre-treated with the essential oil spent longer in the novel arm of Y-maze and with the novel objects in novel object recognition test. The major components in the essential oil, such as thymol, p-cymene and γ-terpinene, could be responsible for the antioxidant activity and inhibition of AChE activity that improve cognitive performance. Devidas et al. tested the effect of 1-Hydroxy-5,5-dimethyl-5,6,7,8-tetrahydro-9,10-anthraquinone, a previously undescribed anthraquinone isolated from walnut ( Juglans regia L.), on the scopolamine-induced cognitive deficit zebrafish model [ 47 ]. The authors found that the compound increased the fish entries and time spent in the correct arm of the T-maze following the scopolamine insult. The protective effect of the compound is likely mediated via cholinergic neurotransmission because the compound exhibited potent inhibition of AChE activity in an in vitro cell-free assay. Many other studies have utilized the scopolamine-induced cognitive impaired model in zebrafish to screen for neuroprotective agents, such as peptides from walnut protein hydrolysates [ 73 ], lithium carbonate (bipolar disorder drug) [ 57 ], sulforaphane (found in crucifereous vegetables) [ 72 ], adenosine signaling-related compounds (caffeine, ZM 241385, DPCPX, dipyridamole and EHNA) [ 70 ], flavonoids (quercetin and rutin) [ 71 ], and physostigmine (AChE inhibitor) [ 53 ].
Lim et al. used a high-cholesterol diet-induced cognitive impairment model of zebrafish to explore the effect of two lactic acid bacteria (LAB) strains: Pediococcus acidilactici LAB4 and Lactobacillus plantarum LAB12 [ 75 ]. The fish in the high-cholesterol diet groups were fed with hard-boiled egg yolk, while the LBAs were incorporated into the feed. Both LABs increased the correct response of high-cholesterol diet-fed fish for food reward in a spatial alternation task. The enhancement of spatial learning was inversely associated with amyloid precursor protein A gene expression. In addition, the LABs reduced body weight and cholesterol in serum and liver. The cholesterol-lowering properties of the LABs were related to the down-regulation of Niemann-Pick C1 Like 1 gene in the intestine that mediates cholesterol uptake, and elevation of ATP-binding cassette family transporter type A1 gene in the liver that regulates cholesterol efflux. This study uncovered the possible use of zebrafish to determine the relationship between a high-fat diet and memory impairment and the therapeutic effects of dietary supplements against the impairment. Zang et al. also demonstrated the neuroprotective effect of probiotics Lactobacillus plantarum ST-III using the triclosan-induced neurodegeneration zebrafish model [ 74 ]. They prepared the probiotics diet by adding the LAB into the feed. The feeding was quantitatively controlled based on the number of fish in the tank. The probiotics diet reduced distance traveled and elevated the number of transitions to the target side in T-maze for triclosan-treated fish. Cognitive improvement by the probiotics was linked to the recovery of the gut microbiome communities, lipid metabolism and intestinal mucosal immunity following triclosan exposure. These studies demonstrate the feasibility of zebrafish as a model to screen for supplements such as probiotics.
Kundap et al. developed a zebrafish model of epilepsy that induced seizure-like behaviors and cognitive dysfunction using pentylenetetrazole (PTZ) [ 80 ]. The model was used in further studies to assess the effect of embelin, a benzoquinone derived from a false black pepper ( Embelia ribes ), on acute and chronic PTZ-induced seizures and cognitive impairment. In the acute seizure model, pre-treatment of embelin (0.078–0.625 mg/kg) reduced seizures and ameliorated epilepsy-associated cognitive dysfunction induced by a single dose (170 mg/kg) of PTZ [ 67 ]. Time spent in the wrong arm and distance traveled by the PTZ-treated fish in the T-maze were decreased by embelin. Neuroprotective mechanisms of embelin may be mediated via the γ-aminobutyric acid (GABA) receptor pathway because the compound showed high affinity toward the receptor in the docking study and increased GABA level in the fish brain. In contrast, a chronic seizure model was induced by a daily dose (80 mg/kg) of PTZ for 10 days [ 68 ]. Daily pre-treatment of embelin (0.156–0.625 mg/kg) reduced the navigation time and performance errors in a three-axis maze test. Embelin increased neurotransmitters (GABA, glutamate, ACh) and showed anti-inflammatory action via the down-regulation of pro-inflammatory gene expressions ( ccl2, tlr-4, tnf-α, il-1, ifn-γ ).
Quercetin has neuroprotective properties but is limited with lower bioavailability and permeability across the blood–brain barrier. Rishitha and Muthiraman studied the efficacy of nanoparticle-formulated quercetin on PTZ-induced cognitive impairment in zebrafish [ 69 ]. Intraperitoneal injection of solid lipid nanoparticle of quercetin at 5 and 10 mg/kg prior to PTZ administration increased the time spent in the preferred chamber and decreased the number of entries to the non-preferred chamber in a two-chamber test. In another test known as the partition preference test, the time spent in and the entry to the target chamber was increased by quercetin-loaded nanoparticle pre-treatment following PTZ induction. The authors also found that quercetin-loaded nanoparticles increased time spent in the upper (preferred) segment and decreased time spent in the lower segment in a three-horizontal compartment test. The neuroprotective effect of quercetin-loaded nanoparticles is presumed to be mediated via their antioxidative properties and neurotransmitter regulation, as the drug increased the reduced glutathione and decreased the thiobarbituric acid reactive substance (a lipid peroxidation marker) and AChE activity in the fish brain.
N-Methyl-D-aspartate receptors are ionotropic glutamate receptors related to cognitive functions. MK-801, an N-Methyl-D-aspartate receptor antagonist, has been shown to induce amnesia in zebrafish [ 81 , 82 , 83 ]. On the other hand, Seibt et al. used MK-801-exposed zebrafish to determine the effect of antipsychotic drugs on neurobehaviors [ 66 ]. Antipsychotic drugs, including sulpiride and haloperidol, restored the cognitive and social interaction deficits in fish exposed to MK-801. The drugs increased the latency to enter the dark compartment with ES in the IAT and the social interaction time in the social preference test.
Alcohol consumption in higher doses can impair cognitive functions leading to amnesia or blackout. Rapid elevated ethanol levels impair memory consolidation, resulting in memory gaps for individuals during intoxication. Bertoncello et al. developed a novel ethanol-induced amnesia model in zebrafish to evaluate the effect of taurine on memory consolidation [ 76 ]. The animals were exposed to taurine for an hour, followed by 1% ethanol for another hour. Both exposures were performed by immersing the fish in beakers. The ethanol-induced deficit in memory consolidation was prevented by taurine. The ethanol-exposed fish pre-treated with taurine had longer latency to enter the punished arm with ES in an IAT. In addition, neuroinflammation triggered by chronic alcohol consumption could lead to cognitive impairment. Rajesh et al. evaluated the effect of mefenamic acid, a non-steroidal anti-inflammatory drug, on a model of chronic alcohol-induced cognitive impairment in zebrafish [ 54 ]. Mefenamic acid decreased the time spent and the number of entries into the dark compartment with water stirring in an IAT for chronic ethanol-exposed fish. AChE activity was reduced in the mefenamic acid-treated group, implying the involvement of the cholinergic anti-inflammatory pathway.
Hypoxia results in ischemic injuries to neurons and cognitive deficits. It inhibits glutamate uptake into the neurons. Urinary trypsin inhibitor (UTI) is a urinary glycoprotein that inhibits proteolytic enzymes such as hyaluronidase plasmin, α-chymotrypsin and trypsin. Kim et al. demonstrated the neuroprotective effect of UTI on a cognitively impaired zebrafish model induced by hypoxia [ 65 ]. UTI-treated fish before hypoxic condition had increased time spent in the target compartment of the T-maze. UTI reduced hypoxia-induced SA-β-galactoside and brain infarction and increased GABA A receptors. Using the hypoxic zebrafish model, the same research group showed that magnesium sulfate prevented cognitive deficits and brain infarction via the up-regulation of EET4A glutamate receptor [ 64 ].
Healthy and cognitively unimpaired zebrafish are models for screening compounds or conditions with memory enhancement (nootropic) properties [ 46 , 84 , 85 , 86 , 87 , 88 , 89 , 90 ] ( Supplementary Table S2 ). Yoo et al. used zebrafish to screen for the nootropic effect of UTI [ 90 ]. UTI increased the time spent, moving distance and frequency of the fish in the target compartment of the T-maze. The cognitive enhancement properties of UTI were related to the up-regulation of the protein level of EET4A glutamate receptor, and gene expressions of c-fos and bdnf in the zebrafish brain. Pusceddu et al. utilized zebrafish to evaluate the chronic effects of Mediterranean natural extracts, namely licorice ( Glycyrrhiza glabra ) root extract and rosemary extract, on cognitive functions [ 86 ]. Both extracts at lower concentrations (100 mg/L) improved the retention memory in the T-maze but induced off-target activity at higher concentrations (250 mg/L). Grossman et al. assessed the effect of piracetam, a drug widely regarded as a nootropic agent, on zebrafish [ 89 ]. The authors showed that acute piracetam exposure at 700 mg/L for 20 min inhibited swimming in a novel tank test. Chronic piracetam exposure at 200 mg/L for 7 days enhanced the cognitive performance by decreasing the latency to the target arm and increasing the duration in and the entries to the target arm. However, the chronic exposure increased total (correct and incorrect) arm entries, indicating the hypermobility effect of the drug. Echevarria et al. tested the memory-enhancing effect of methylene blue using healthy zebrafish [ 85 ]. Methylene blue showed a hormetic dose–response curve (U-shaped curve with a biphasic dose response) such that fish treated with 10 μM (high dose) methylene blue via immersion performed worse than the compound at 0.5 and 5 μM (low dose) in the T-maze. Nicotine and nicotinic agonists could enhance cognitive performance via α7 and α4β2 nicotinic acetylcholine receptors (nAChRs). Levin and Chen utilized zebrafish to determine the nicotinic involvement in learning and memory functions [ 88 ]. Acute exposure (3 min) to nicotine exhibited a biphasic effect on memory function, such that the fish performed better at a low dose (<100 mg/L) and displayed poor performance at a high dose (>100 mg/L) in an IAT. A further study from the same group found that the cognitive enhancement effect of nicotine at 100 mg/L was the most effective at 20–40 min post-administration [ 87 ]. Braida et al. leveraged the reward pathway in zebrafish to screen for newly developed α4β2 nAChR ligands as psychoactive compounds [ 46 ]. They found that the full agonists MCL-11 and MCL-28 enhanced the spatial and recognition memory of fish in an inverted U-shaped dose response. Based on the binding assay, MCL-11 was more potent than nicotine to zebrafish nAChRs. MCL-11 at 0.01 g/kg and MCL-28 at 1 g/kg administered intraperitoneally 20 min before the probe phase in the T-maze decreased the latency to reach the targeted arm. Such effects were blocked by the antagonist MCL-117, reinforcing the specific actions of these ligands on nAChRs.
4.2. Neurotoxicity Screening
Zebrafish are a popular model used to screen for compounds with neurotoxicity effects on learning and memory. These include metals/elements [ 48 , 52 , 91 , 92 , 93 , 94 , 95 , 96 , 97 , 98 , 99 , 100 , 101 ], environmental pollutants [ 102 , 103 , 104 , 105 , 106 , 107 ], stimulant drugs [ 108 , 109 , 110 , 111 , 112 ], alcohol [ 42 , 113 , 114 ] and others [ 81 , 82 , 83 , 115 , 116 , 117 , 118 , 119 , 120 , 121 , 122 , 123 , 124 , 125 ]. ( Supplementary Table S3 ). Lower maintenance costs and the availability of diverse behavioral assays for zebrafish are the main reasons to conduct neurotoxicity screening using this animal model. The findings could be translated into human exposure or used to validate the mechanisms involved.
Shang et al. used zebrafish to validate the neurotoxic mechanism of occupational aluminum exposure in humans [ 92 ]. Serum aluminum in occupationally exposed workers was inversely correlated with cognitive score and the PI3K/Akt/mTOR signaling pathway. These findings were corroborated in aluminum-exposed zebrafish. Increased aluminum in the zebrafish brain was associated with impaired learning and memory performance and reduced PI3K/Akt/mTOR expression. Zebrafish housed in tank water containing aluminum trichloride for 30 days had increased latency to enter and decreased cumulative time in the targeted zone of T-maze. Similarly, Chen et al. examined the progressive effect of long-term accumulation of aluminum oxide nanoparticles on the learning and memory functions of zebrafish from embryo to adult [ 48 ]. In the study, 6 h post fertilization (hpf) embryos were exposed to the nanoparticles until 120 hpf and subjected to behavioral assays at 6 to 12 months old. Chronic embryonic exposure to the nanoparticles impaired the learning and memory performance of zebrafish in adulthood. Zebrafish exposed to the nanoparticles had a longer tendency to enter and lesser cumulative time in the targeted zone in the T-maze. The nanoparticle-induced oxidative stress might be responsible for the reduced neurotransmitters (ACh and dopamine) in the brain and the induction of neural cell death and autophagy in the telencephalon region of the zebrafish brain.
Bui Thi et al. utilized zebrafish to evaluate the effect of chronic lead exposure on neurobehaviors [ 101 ]. Low levels (50 ppb) of lead chloride (PbCl 2 ) reduced the fish locomotor activity and aggressive behaviors in a 3D LAT and mirror biting test, respectively. In the mirror biting test, a mirror was located next to the tank. The aggressive behavior was scored based on biting and fast swimming, as zebrafish tend to display boldness by biting the mirror and tracing their reflection by quick movement. The PbCl 2 induced anxiety-like behaviors in a novel tank test and altered circadian rhythm locomotor activity. Fish exposed to PbCl 2 had reduced latency to enter the dark compartment with ES in the IAT, indicating memory loss induced by PbCl 2 poisoning. Elevation of cortisol and reduction of serotonin and melatonin in the brain could be related to the altered neurobehaviors observed in PbCl 2 -exposed zebrafish. Sarasamma et al. developed an AD model based on zinc chloride (ZnCl 2 )-induced cognitive impairment in zebrafish [ 52 ]. ZnCl 2 decreased the latency to enter the dark chamber with ES in an IAT for trained fish, signifying lower memory retention. Elevation of reactive oxygen species level, lipid peroxidation markers (malondialdehyde, thiobarbituric acid reactive substance and 4-hydroxynonenal) and stress hormones (catecholamine and cortisol) and reduction of melatonin and antioxidant enzyme activities (the reduced glutathione, glutathione peroxidase and superoxide dismutase) were observed in the brain following ZnCl 2 exposure. The compound also affected neurotransmitters by increasing AChE activity, dopamine, glutamate and GABA levels, while decreasing glycine and histidine levels. More importantly, ZnCl 2 -exposed zebrafish displayed AD-like symptoms by elevating amyloid beta 42 and phosphorylated tau proteins in the brain.
Audira et al. investigated the adverse effect of chronic exposure to donepezil on cognitive functions and behaviors, using normal zebrafish as a model to represent healthy individuals without AD [ 125 ]. Long-term exposure to 2.5 ppm donepezil for 21 days reduced time spent in the punished arm in the T-maze for the trained fish, suggesting the memory enhancement effect of donepezil. The altered behaviors were associated with increased oxytocin and reduced cortisol in the brain. Although donepezil exhibited anxiolytic properties, it reduced locomotor activity and elevated aggressive behavior. An increments of reactive oxygen species and malondialdehyde in the muscle could underlie the locomotor impairment induced by donepezil. Therefore, the long-term usage of donepezil should be cautious because chronic exposure might have an adverse effect on normal individuals even though it improves memory retention.
Zebrafish larvae can be used to perform toxicity screening on learning and memory. He et al. used zebrafish larvae to investigate the effect of prenatal exposure to propofol, a widely used general anesthetic drug, on learning and memory function [ 118 ]. Propofol exposure at 6 until 48 hpf spared the spontaneous locomotor activity of the larvae but diminished the “activity decrease” in a dark-to-light cycle test. “Activity decrease” represents the response to repeated photoperiod stimulus, and the decline is considered as habituation learning exhibited by the larvae. The authors also found that propofol-induced cognitive impairment was correlated to the inhibition of axonal growth in motor neurons. Hu et al. investigated the joint effects of two pesticides (chlorpyrifos and deltamethrin) on the zebrafish larvae by co-exposing both compounds to the embryo at 2 until 144 hpf [ 123 ]. Co-exposure to the pesticides increased mortality and malformation rates, while decreased the hatching rate of the embryos. The co-exposure decreased swimming speed and adaptability to repeated stimulation in a dark-to-light cycles test, representing a deficit in habituation learning for the larvae. The co-exposure also altered metabolisms of glycerophospholipids and amino acids.
5. Limitations of Zebrafish as a Pharmacological Model for Learning and Memory Research
There are several concerns when using zebrafish as models for drug discovery in cognitive research. First, aqueous immersion was the major route of drug administration used in many studies, which might be least applicable to humans. Aqueous immersion was chosen probably due to the convenient setup. However, the bioavailability of the drugs is difficult to assess in zebrafish via this method. Over the years, other routes of administration, such as intraperitoneal [ 65 , 67 , 122 ], oral administration [ 64 ] and cerebroventricular injection [ 56 , 120 ] have been reported, which have greater translation value. The second limitation is the throughput of a behavioral test for drug screening. Zebrafish are social animals that prefer to swim in a group. Cognitive testing often requires the animal to be assessed individually, but this may incur anxiety and stress responses in the zebrafish. A longer habitation session could overcome this problem but greatly reduces the assay throughout when involving a large number of fish for drug screening. Valente et al. developed an automated platform that used an LCD screen to present the visual cues to the larvae in a group [ 31 ]. An automated setup that can run the paradigms in parallel and track them simultaneously by software have been developed. The software tracks multiple zebrafish simultaneously either as an individual in a multi-well plate or as a group in a tank. There are tools/software available for high-throughput screening of larval (mostly) and adult zebrafish behaviors, but they are mainly limited to locomotion and social behaviors [ 45 ]. These software include idTracker (trajectories, locomotor activity, social behaviors) [ 126 ], Multi-Animal Tracker (locomotor activity) [ 127 ], ToxTrac (locomotor activity) [ 128 ] and TrackId (new algorithm implemented on ToxTrac that decreased recognition error rate) [ 129 ]. Further development of the algorithm is necessary to encompass learning paradigms, for example, to automatically stop tracking individual zebrafish that have completed the conditioning in a group or parallel assay.
6. Future Perspectives
In this review, we have seen that zebrafish have emerged as one of the vertebrate models for cognitive research related to learning and memory functions over the past decade. The gaining popularity is supported by a repertoire of neurobehavioral displayed by the zebrafish and assessment tools available for these behaviors. Zebrafish have been proven useful as pharmacological models in drug screening for neuroprotective or neurotoxicity agents that affect learning and memory performance. Zebrafish emerged as a complementary model to rodents in preclinical studies before further testing on humans. One of the main advantages of the zebrafish model is lower maintenance costs for pharmacological screening. However, the clinically relevant routes of drug administration still require further exploration. In addition, innovative behavioral assessment apparatuses and software are needed to increase the assay throughput. By overcoming these limitations and combining the favorable features of zebrafish, this animal model will be indispensable in drug discovery research involving learning and memory.
Supplementary Materials
The following supporting information can be downloaded at: https://www.mdpi.com/article/10.3390/molecules27217374/s1 , Table S1: Cognitive impairment models of zebrafish and pharmacological studies for compounds with neuroprotective effects; Table S2: Screening for nootropic agents with memory-enhancing properties using zebrafish as model; Table S3: Neurotoxicity studies for compounds that affect learning and memory in zebrafish.
Funding Statement
This work was funded by Universiti Kebangsaan Malaysia, grant number GUP-2018-056.
Author Contributions
Conceptualization, J.K.T.; writing—original draft preparation, J.K.T. and F.H.N.; writing—review and editing, J.K.T., F.H.N., S.M. and S.L.T.; supervision, J.K.T.; project administration, J.K.T.; funding acquisition, J.K.T. All authors have read and agreed to the published version of the manuscript.
Institutional Review Board Statement
Informed consent statement, data availability statement, conflicts of interest.
The authors declare no conflict of interest. The funders had no role in the design of the study; in the collection, analyses, or interpretation of data; in the writing of the manuscript; or in the decision to publish the results.
Publisher’s Note: MDPI stays neutral with regard to jurisdictional claims in published maps and institutional affiliations.
Thank you for visiting nature.com. You are using a browser version with limited support for CSS. To obtain the best experience, we recommend you use a more up to date browser (or turn off compatibility mode in Internet Explorer). In the meantime, to ensure continued support, we are displaying the site without styles and JavaScript.
- View all journals
- Explore content
- About the journal
- Publish with us
- Sign up for alerts
- Review Article
- Published: 15 June 2020
Zebrafish microbiome studies make waves
- Keaton Stagaman ORCID: orcid.org/0000-0003-2815-4530 1 ,
- Thomas J. Sharpton 1 , 2 &
- Karen Guillemin ORCID: orcid.org/0000-0001-6004-9955 3 , 4
Lab Animal volume 49 , pages 201–207 ( 2020 ) Cite this article
2127 Accesses
43 Citations
20 Altmetric
Metrics details
- Ichthyology
Zebrafish have a 50-year history as a model organism for studying vertebrate developmental biology and more recently have emerged as a powerful model system for studying vertebrate microbiome assembly, dynamics and function. In this Review, we discuss the strengths of the zebrafish model for both observational and manipulative microbiome studies, and we highlight some of the important insights gleaned from zebrafish gut microbiome research.
This is a preview of subscription content, access via your institution
Access options
Subscribe to this journal
We are sorry, but there is no personal subscription option available for your country.
Rent or buy this article
Prices vary by article type
Prices may be subject to local taxes which are calculated during checkout
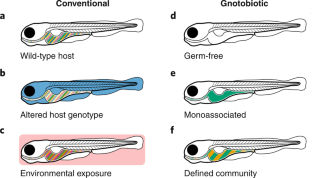
Similar content being viewed by others
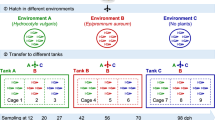
Host development overwhelms environmental dispersal in governing the ecological succession of zebrafish gut microbiota
Fanshu Xiao, Wengen Zhu, … Qingyun Yan
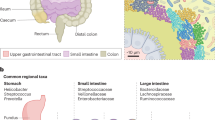
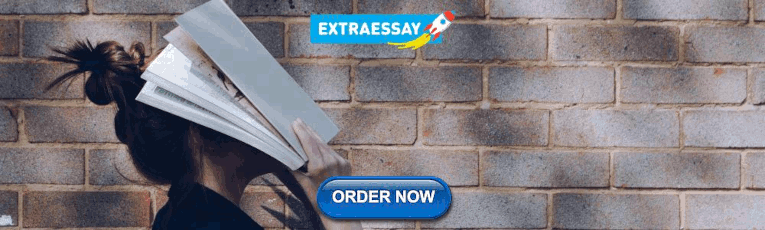
The gut microbiota and its biogeography
Giselle McCallum & Carolina Tropini
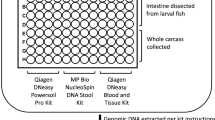
Experimental methods modestly impact interpretation of the effect of environmental exposures on the larval zebrafish gut microbiome
Keaton Stagaman, Kristin D. Kasschau, … Thomas J. Sharpton
Grunwald, D. J. & Eisen, J. S. Headwaters of the zebrafish—emergence of a new model vertebrate. Nat. Rev. Genet. 3 , 717–724 (2002).
Article CAS PubMed Google Scholar
Burns, A. R. & Guillemin, K. The scales of the zebrafish: host–microbiota interactions from proteins to populations. Curr. Opin. Microbiol. 38 , 137–141 (2017).
Article CAS PubMed PubMed Central Google Scholar
Stephens, W. Z. et al. The composition of the zebrafish intestinal microbial community varies across development. ISME J. 10 , 644–654 (2016).
Article PubMed CAS Google Scholar
Gaulke, C. A., Barton, C. L., Proffitt, S., Tanguay, R. L. & Sharpton, T. J. Triclosan exposure is associated with rapid restructuring of the microbiome in adult zebrafish. PLoS One 11 , e0154632 (2016).
Article PubMed PubMed Central CAS Google Scholar
Hill, J. H., Franzosa, E. A., Huttenhower, C. & Guillemin, K. A conserved bacterial protein induces pancreatic beta cell expansion during zebrafish development. Elife 5 , e20145 (2016).
Article PubMed PubMed Central Google Scholar
Rolig, A. S. et al. A bacterial immunomodulatory protein with lipocalin-like domains facilitates host–bacteria mutualism in larval zebrafish. Elife 7 , e37172 (2018).
Rawls, J. F., Samuel, B. S. & Gordon, J. I. Gnotobiotic zebrafish reveal evolutionarily conserved responses to the gut microbiota. Proc. Natl Acad. Sci. USA 101 , 4596–4601 (2004).
Bates, J. M. et al. Distinct signals from the microbiota promote different aspects of zebrafish gut differentiation. Dev. Biol. 297 , 374–386 (2006).
Melancon, E. et al. Best practices for germ-free derivation and gnotobiotic zebrafish husbandry. Methods Cell Biol. 138 , 61–100 (2017).
Lewis, K. L., Del Cid, N. & Traver, D. Perspectives on antigen presenting cells in zebrafish. Dev. Comp. Immunol. 46 , 63–73 (2014).
Stednitz, S. J. et al. Forebrain control of behaviorally driven social orienting in zebrafish. Curr. Biol. 28 (2445-2451), e3 (2018).
Google Scholar
Davis, D. J. et al. Lactobacillus plantarum attenuates anxiety-related behavior and protects against stress-induced dysbiosis in adult zebrafish. Sci. Rep. 6 , 33726 (2016).
Borrelli, L. et al. Probiotic modulation of the microbiota-gut-brain axis and behaviour in zebrafish. Sci. Rep. 6 , 30046 (2016).
Truong, L. et al. Multidimensional in vivo hazard assessment using zebrafish. Toxicol. Sci. 137 , 212–233 (2014).
Phelps, D. et al. Microbial colonization is required for normal neurobehavioral development in zebrafish. Sci. Rep. 7 , 11244 (2017).
Bates, J. M., Akerlund, J., Mittge, E. & Guillemin, K. Intestinal alkaline phosphatase detoxifies lipopolysaccharide and prevents inflammation in zebrafish in response to the gut microbiota. Cell Host Microbe 2 , 371–382 (2007).
Cheesman, S. E., Neal, J. T., Mittge, E., Seredick, B. M. & Guillemin, K. Epithelial cell proliferation in the developing zebrafish intestine is regulated by the Wnt pathway and microbial signaling via Myd88. Proc. Natl Acad. Sci. USA 108 , 4570–4577 (2011).
Troll, J. V. et al. Microbiota promote secretory cell determination in the intestinal epithelium by modulating host Notch signaling. Development 145 , dev155317 (2018).
Murdoch, C. C. & Rawls, J. F. Commensal microbiota regulate vertebrate innate immunity—insights from the zebrafish. Front. Immunol. 10 , 2100 (2019).
Ye, L. et al. High fat diet induces microbiota-dependent silencing of enteroendocrine cells. Elife 8 , e48479 (2019).
Semova, I. et al. Microbiota regulate intestinal absorption and metabolism of fatty acids in the zebrafish. Cell Host Microbe 12 , 277–288 (2012).
Davis, D. J., Bryda, E. C., Gillespie, C. H. & Ericsson, A. C. Microbial modulation of behavior and stress responses in zebrafish larvae. Behav. Brain Res. 311 , 219–227 (2016).
Bosch, T. C. G., Guillemin, K. & McFall-Ngai, M. Evolutionary ‘experiments’ in symbiosis: the study of model animals provides insights into the mechanisms underlying the diversity of host-microbe interactions. Bioessays 41 , e1800256 (2019).
Wiles, T. J. et al. Modernized tools for streamlined genetic manipulation and comparative study of wild and diverse proteobacterial lineages. mBio 9 , e01877–18 (2018).
Taormina, M. J. et al. Investigating bacterial-animal symbioses with light sheet microscopy. Biol. Bull. 223 , 7–20 (2012).
Jemielita, M. et al. Spatial and temporal features of the growth of a bacterial species colonizing the zebrafish gut. mBio 5 , e01751–14 (2014).
Wiles, T. J. et al. Host gut motility promotes competitive exclusion within a model intestinal microbiota. PLoS Biol. 14 , e1002517 (2016).
Schlomann, B. H., Wiles, T. J., Wall, E. S., Guillemin, K. & Parthasarathy, R. Sublethal antibiotics collapse gut bacterial populations by enhancing aggregation and expulsion. Proc. Natl Acad. Sci. USA 116 , 21392–21400 (2019).
Wiles, T. J. et al. Swimming motility of a gut bacterial symbiont promotes resistance to intestinal expulsion and enhances inflammation. PLoS Biol. 18 , e3000661 (2020).
Schlomann, B. H., Wiles, T. J., Wall, E. S., Guillemin, K. & Parthasarathy, R. Bacterial cohesion predicts spatial distribution in the larval zebrafish intestine. Biophys. J. 115 , 2271–2277 (2018).
Stephens, W. Z. et al. Identification of population bottlenecks and colonization factors during assembly of bacterial communities within the zebrafish intestine. mBio 6 , e01163–15 (2015).
Bae, S., Mueller, O., Wong, S., Rawls, J. F. & Valdivia, R. H. Genomic sequencing-based mutational enrichment analysis identifies motility genes in a genetically intractable gut microbe. Proc. Natl Acad. Sci. USA 113 , 14127–14132 (2016).
Rawls, J. F., Mahowald, M. A., Ley, R. E. & Gordon, J. I. Reciprocal gut microbiota transplants from zebrafish and mice to germ-free recipients reveal host habitat selection. Cell 127 , 423–433 (2006).
Burns, A. R. et al. Interhost dispersal alters microbiome assembly and can overwhelm host innate immunity in an experimental zebrafish model. Proc. Natl Acad. Sci. USA 114 , 11181–11186 (2017).
Stagaman, K., Burns, A. R., Guillemin, K. & Bohannan, B. J. The role of adaptive immunity as an ecological filter on the gut microbiota in zebrafish. ISME J. 11 , 1630–1639 (2017).
Roeselers, G. et al. Evidence for a core gut microbiota in the zebrafish. ISME J. 5 , 1595–1608 (2011).
Spence, R., Gerlach, G., Lawrence, C. & Smith, C. The behaviour and ecology of the zebrafish, Danio rerio. Biol. Rev. Camb. Philos. Soc. 83 , 13–34 (2008).
Article PubMed Google Scholar
Wong, S. et al. Ontogenetic differences in dietary fat influence microbiota assembly in the zebrafish gut. mBio 6 , e00687–15 (2015).
Givens, C., Ransom, B., Bano, N. & Hollibaugh, J. Comparison of the gut microbiomes of 12 bony fish and 3 shark species. Mar. Ecol. Prog. Ser. 518 , 209–223 (2015).
Article Google Scholar
Egerton, S., Culloty, S., Whooley, J., Stanton, C. & Ross, R. P. The gut microbiota of marine fish. Front. Microbiol. 9 , 873 (2018).
Ding, Q. et al. The hepatotoxicity of palmitic acid in zebrafish involves the intestinal microbiota. J. Nutr. 148 , 1217–1228 (2018).
Guo, X. et al. The growth-promoting effect of dietary nucleotides in fish is associated with an intestinal microbiota-mediated reduction in energy expenditure. J. Nutr. 147 , 781–788 (2017).
Teame, T. et al. The use of zebrafish ( Danio rerio ) as biomedical models. Anim. Front. 9 , 68–77 (2019).
Burns, A. R. et al. Contribution of neutral processes to the assembly of gut microbial communities in the zebrafish over host development. ISME J. 10 , 655–664 (2016).
Brito, I. L. & Alm, E. J. Tracking strains in the microbiome: insights from metagenomics and models. Front. Microbiol. 7 , 712 (2016).
PubMed PubMed Central Google Scholar
Merrifield, D. L. et al. Ingestion of metal-nanoparticle contaminated food disrupts endogenous microbiota in zebrafish ( Danio rerio ). Environ. Pollut. 174 , 157–163 (2013).
Catron, T. R. et al. Host developmental toxicity of BPA and BPA alternatives is inversely related to microbiota disruption in zebrafish. Toxicol. Sci. 167 , 468–483 (2019).
Liu, Y. et al. Influence of endogenous and exogenous estrogenic endocrine on intestinal microbiota in zebrafish. PLoS One 11 , e0163895 (2016).
Zang, L. et al. Dietary Lactobacillus plantarum ST-III alleviates the toxic effects of triclosan on zebrafish ( Danio rerio ) via gut microbiota modulation. Fish Shellfish Immunol. 84 , 1157–1169 (2019).
Gaulke, C. A. et al. A longitudinal assessment of host-microbe-parasite interactions resolves the zebrafish gut microbiome’s link to Pseudocapillaria tomentosa infection and pathology. Microbiome 7 , 10 (2019).
Paquette, C. E. et al. A retrospective study of the prevalence and classification of intestinal neoplasia in zebrafish ( Danio rerio ). Zebrafish 10 , 228–236 (2013).
Burns, A. R. et al. Transmission of a common intestinal neoplasm in zebrafish by cohabitation. J. Fish Dis. 41 , 569–579 (2018).
Rolig, A. S. et al. The enteric nervous system promotes intestinal health by constraining microbiota composition. PLoS Biol. 15 , e2000689 (2017).
Ran, C. et al. Aeromonas veronii and aerolysin are important for the pathogenesis of motile aeromonad septicemia in cyprinid fish. Environ. Microbiol. 20 , 3442–3456 (2018).
Download references
Acknowledgements
This work was supported in part by grants from the National Institutes of Health, under P01GM125576 to K.G., the National Institutes of Environmental Health Sciences (1R01ES030226) to T.J.S. K.S. was supported in part by the NIEHS Integrated Regional Training Program in Environmental Health Sciences grant (PI R.L. Tanguay, T32-ES007060-38).
Author information
Authors and affiliations.
Department of Microbiology, Oregon State University, Corvallis, OR, USA
Keaton Stagaman & Thomas J. Sharpton
Department of Statistics, Oregon State University, Corvallis, OR, USA
- Thomas J. Sharpton
Institute of Molecular Biology, University of Oregon, Eugene, OR, USA
Karen Guillemin
Humans and the Microbiome Program, CIFAR, Toronto, Ontario, Canada
You can also search for this author in PubMed Google Scholar
Corresponding author
Correspondence to Karen Guillemin .
Ethics declarations
Competing interests.
The authors declare no competing interests.
Additional information
Publisher’s note Springer Nature remains neutral with regard to jurisdictional claims in published maps and institutional affiliations.
Rights and permissions
Reprints and permissions
About this article
Cite this article.
Stagaman, K., Sharpton, T.J. & Guillemin, K. Zebrafish microbiome studies make waves. Lab Anim 49 , 201–207 (2020). https://doi.org/10.1038/s41684-020-0573-6
Download citation
Received : 14 March 2019
Accepted : 18 May 2020
Published : 15 June 2020
Issue Date : July 2020
DOI : https://doi.org/10.1038/s41684-020-0573-6
Share this article
Anyone you share the following link with will be able to read this content:
Sorry, a shareable link is not currently available for this article.
Provided by the Springer Nature SharedIt content-sharing initiative
This article is cited by
Anti-diarrheal drug loperamide induces dysbiosis in zebrafish microbiota via bacterial inhibition.
- Rebecca J. Stevick
- Bianca Audrain
- David Pérez-Pascual
Microbiome (2023)
Disentangling the link between zebrafish diet, gut microbiome succession, and Mycobacterium chelonae infection
- Michael J. Sieler
- Colleen E. Al-Samarrie
Animal Microbiome (2023)
Challenges and opportunities in sharing microbiome data and analyses
- Curtis Huttenhower
- Robert D. Finn
- Alice Carolyn McHardy
Nature Microbiology (2023)
Zebrafish: an efficient vertebrate model for understanding role of gut microbiota
- Huimin Chen
- Hongtao Liu
Molecular Medicine (2022)
- Keaton Stagaman
- Kristin D. Kasschau
Scientific Reports (2022)
Quick links
- Explore articles by subject
- Guide to authors
- Editorial policies
Sign up for the Nature Briefing newsletter — what matters in science, free to your inbox daily.

Behaviour and morphology of the zebrafish, danio rerio
--> Pritchard, Victoria Louise (2001) Behaviour and morphology of the zebrafish, danio rerio. PhD thesis, University of Leeds.
Speciation remains a process that is poorly understood. In particular, little progress has been made in elucidating the mechanisms underlying prezygotic isolation. A full understanding requires a knowledge of underlying genetics. To date the only real progress has been made using Drosophila: more model organisms are needed. It is proposed that the zebrafish, Danio rerio, a species well suited to the laboratory environment and with much information available about its genetics, has potential in this area. However, currently little is know about mate choice in this species and whether its behaviour and morphology exhibit any genetic variation in the wild that may be amenable to further analysis. This thesis addresses these questions by investigating the behaviour and morphology of zebrafish deriving from several wild populations in Nepal and Bangladesh. Mate choice trials in the laboratory revealed several features of the zebrafish that make it less amenable to such studies than several alternative teleost species. These include a lack of strong sexual dimorphism and the absence of behaviours that reliably indicate mate choice. Nevertheless observations in the course of this study suggest that male carotenoid coloration, longitudinal melanophore stripes and symmetry of caudal fin pattern may have behavioural roles in this species. Investigations into the shoaling behaviour of the zebrafish revealed no evidence for association preferences for familiar individuals or kin. The ubiquity of a preference for familiars in shoaling fish may have been over emphasized. This study did, however, find evidence for inter-population variation in grouping behaviour that may provide another avenue for the investigation of the genetics of behavioural adaptations. Documentation of morphological variation revealed heritable inter-population variability, in particular in body stripe pattern, as well as consistent sexual dimorphisms in body shape and anal fin morphology. Further investigation of the quantitative genetic basis of these traits revealed that several features of body stripe pattern were not heritable under laboratory conditions. This may indicate stabilising selection on body stripe pattern. In contrast, elements of anal fin morphology exhibited more underlying genetic variation.
--> uk_bl_ethos_414524 --> -->
Filename: uk_bl_ethos_414524.pdf
Embargo Date:
You do not need to contact us to get a copy of this thesis. Please use the 'Download' link(s) above to get a copy. You can contact us about this thesis . If you need to make a general enquiry, please see the Contact us page.

There’s a powerful story behind every headline at Ohio State Health & Discovery. As one of the largest academic health centers and health sciences campuses in the nation, we are uniquely positioned with renowned experts covering all aspects of health, wellness, science, research and education. Ohio State Health & Discovery brings this expertise together to deliver today’s most important health news and the deeper story behind the most powerful topics that affect the health of people, animals, society and the world. Like the science and discovery news you find here? You can support more innovations fueling advances across medicine, science, health and wellness by giving today.
BROUGHT TO YOU BY
- The Ohio State University
- College of Dentistry
- College of Medicine
- College of Nursing
- College of Optometry
- College of Pharmacy
- College of Public Health
- College of Veterinary Medicine
- Ohio State Wexner Medical Center
- Ohio State's Comprehensive Cancer Center – James Cancer Hospital and Solove Research Institute
Subscribe. The latest from Ohio State Health & Discovery delivered right to your inbox.
The scientific wonders of zebrafish for biomedical research
Translucent living laboratories let scientists see life processes unfold before their eyes.
Contributing Writer Ohio State Wexner Medical Center
- Share on Facebook
- Share on Linkedin
- Share via Email
- Share this page
The next time you visit an aquarium, look closely at the zebrafish. Those striped swimmers, just an inch or two long, might not look like it, but they carry the potential to revolutionize cancer treatment, enable humans to grow new heart tissue and contribute to countless other medical and scientific breakthroughs.
Zebrafish, or Danio rerio, live naturally in warm, shallow streams and rice paddies where India, Bangladesh and Nepal come together. To the fish hobbyist, they make an attractive and low-maintenance addition to the home tank.
But at The Ohio State University Wexner Medical Center, zebrafish are helping scientists push the boundaries of molecular genetics — revealing secrets and answering questions that could lead to innovations and therapies yet unimagined.
From its origins at the University of Oregon more than 40 years ago, the zebrafish research model has spread worldwide, and Ohio State, with six independent zebrafish labs across campus, is a major player.
“We have several groups using the model to study related aspects of development and disease,” says Sharon Amacher, PhD , a professor with appointments in the College of Arts and Sciences’ Department of Molecular Genetics and the College of Medicine’s Department of Biological Chemistry and Pharmacology. “We really synergize. We meet regularly to share our research.”
Each of Ohio State’s zebrafish labs has a roster of between four and 12 people, for a total of about 50 researchers actively engaged in the work.
Three nurseries, six labs and endless genetic possibilities
Though zebrafish don’t look much like humans, it turns out we’re put together the same in most of the ways that count, with brains, spinal circuits, major organs and about 70% of our DNA in common. Even better, if you consider only the human genes known to be implicated in disease, 84% of those have zebrafish equivalents. And zebrafish have further benefits as a research model: They breed quickly and easily, and they’re small enough to house in large numbers but large enough to allow medical procedures like transplantation. Another distinctive feature? The embryos are transparent, allowing researchers with powerful microscopes to see cell processes unfold in real time. Even zebrafish adults can be made transparent when they carry mutations in two key pigmentation genes.
“In the early days, the model attracted a lot of biologists who wanted to understand how an embryo is put together and how embryos develop, how cells know what to do and when to do it,” Dr. Amacher says.
“But now, zebrafish are great for other studies, too. They get the same diseases humans do, so they’re very important in cancer studies,” Dr. Amacher says.
They’re also amazing at regeneration — a superpower that could have stunning implications for humans. For instance, when a human has a heart attack, those heart cells die and are never replaced. With zebrafish, 50% of the muscle cells of their heart can be destroyed and they will regenerate them within a month or two.
Zebrafish regeneration doesn’t stop with heart muscles. When a zebrafish spinal cord is severed, researchers say, that same fish will be swimming around in about six weeks, indistinguishable from their tankmates.
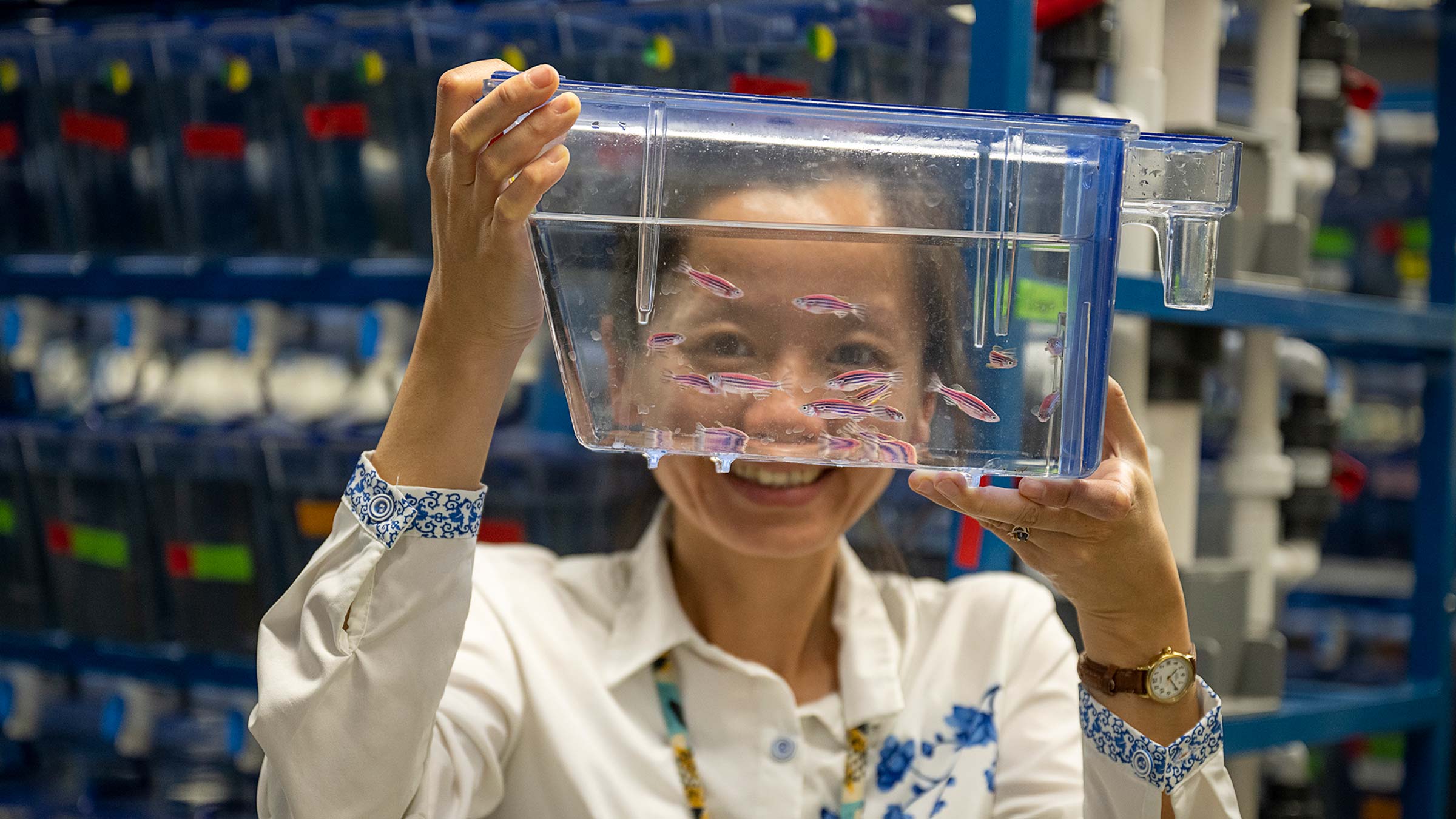
Those tankmates coexist by the thousands in three separate facilities on the medical center campus. Each is a sort of living genetic library, with floor-to-ceiling racks of 3- to 9-liter plastic tanks that are temperature controlled, automatically aerated, and recirculated several times per hour. The fish are grouped by age and genetic makeup, for cultivation of whatever traits are desired by the researchers.
In one campus facility, Dr. Amacher’s lab studies skeletal muscle and vertebral patterning, using the zebrafish as a model for vertebrates (animals that, like us, have spinal columns).
In another fish facility, the transparency of zebrafish embryos allows Martin Haesemeyer, PhD , assistant professor in the Department of Neuroscience at the College of Medicine, to actually see cells at work. Rather than employing electrodes to record electrical activity in neurons, he uses fluorescent proteins that report a change in a cell’s calcium level. This allows Dr. Haesemeyer to directly observe the cell growing brighter or dimmer with each change in activity.
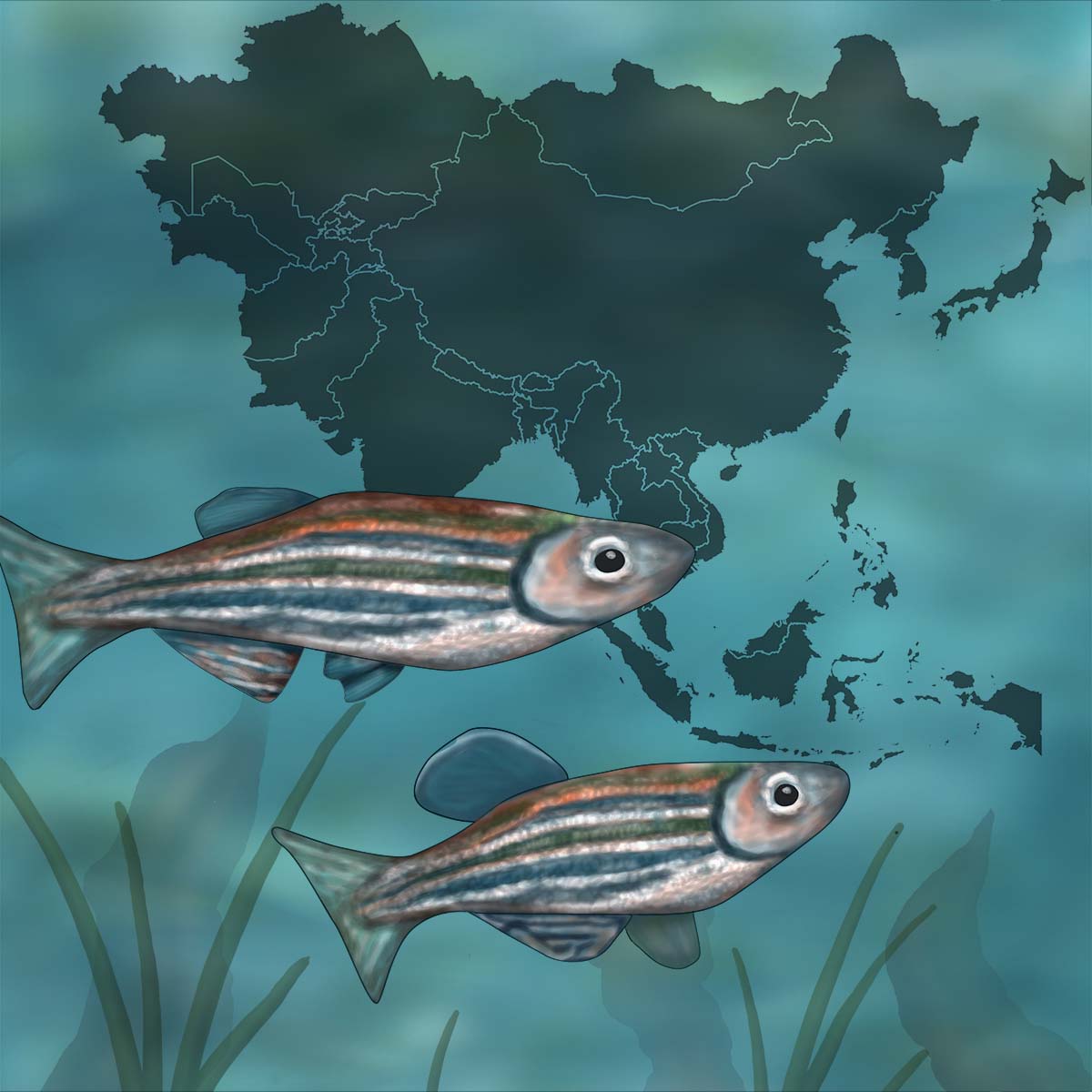
Research possibilities
Zebrafish live in warm, shallow streams in Asia. For medical researchers, this fish holds marvelous abilities.
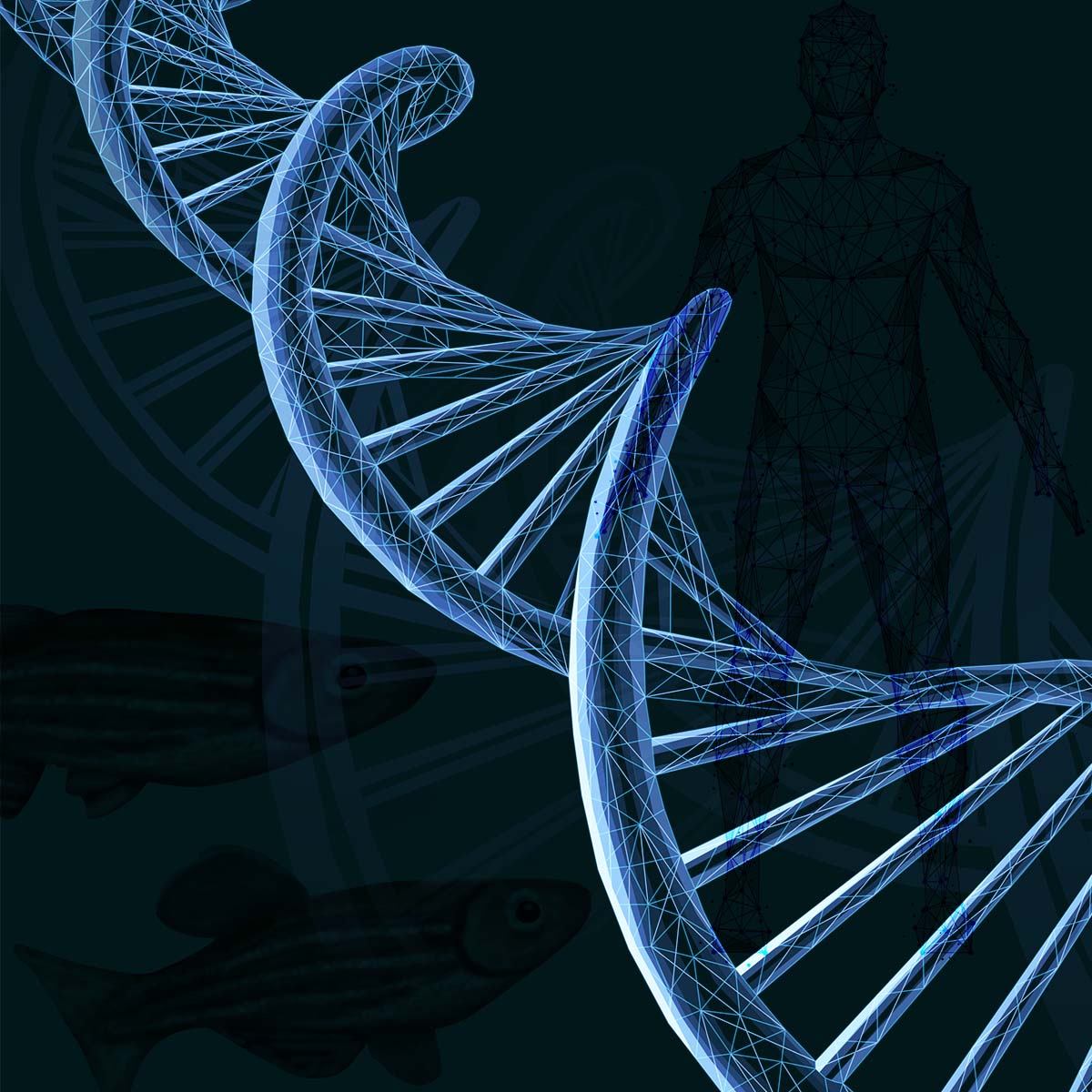
Zebrafish and humans have a lot in common, including organs, spines and roughly 70% of the same DNA.
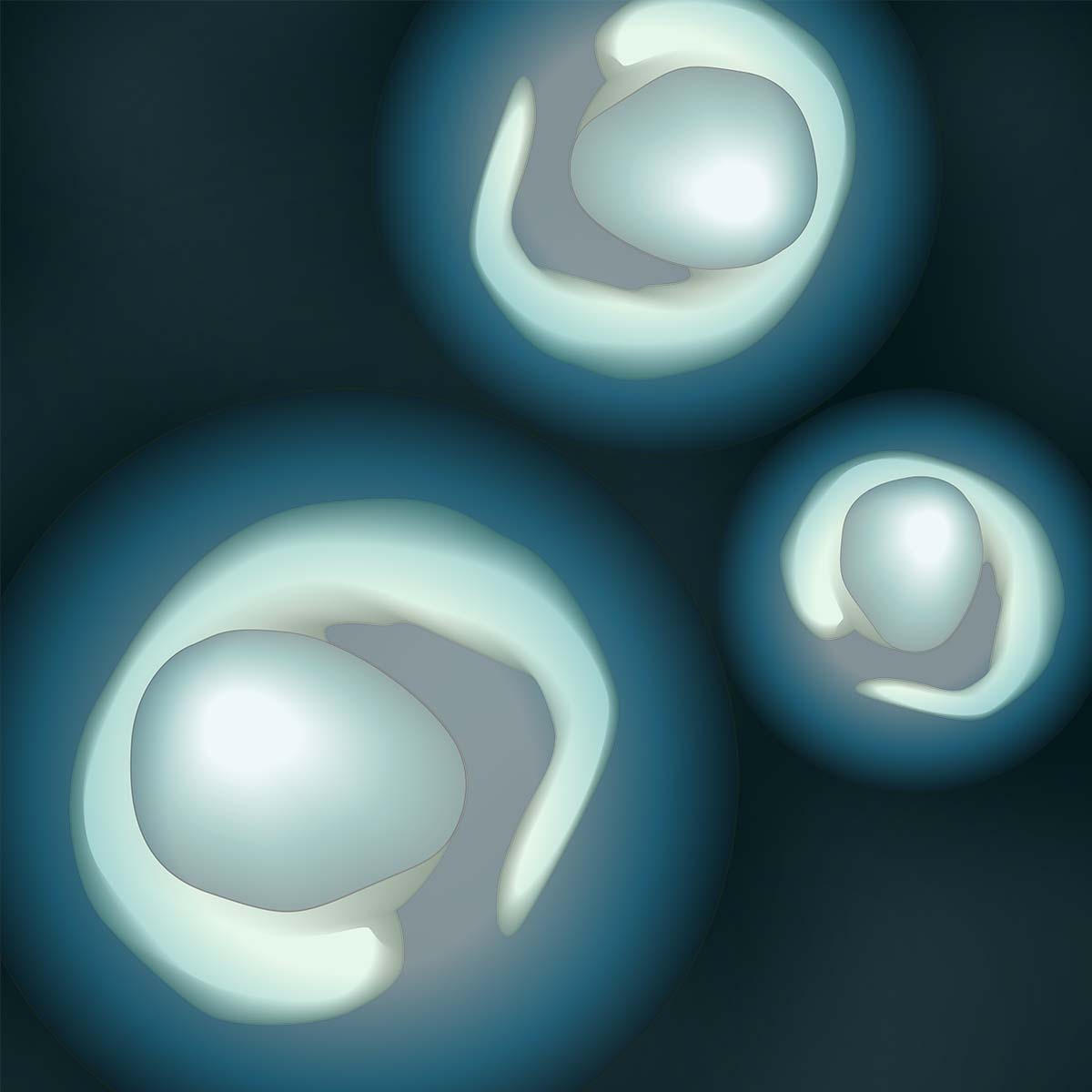
Translucent skin
Zebrafish embryos are translucent, allowing scientists to watch medical processes unfold before their eyes.
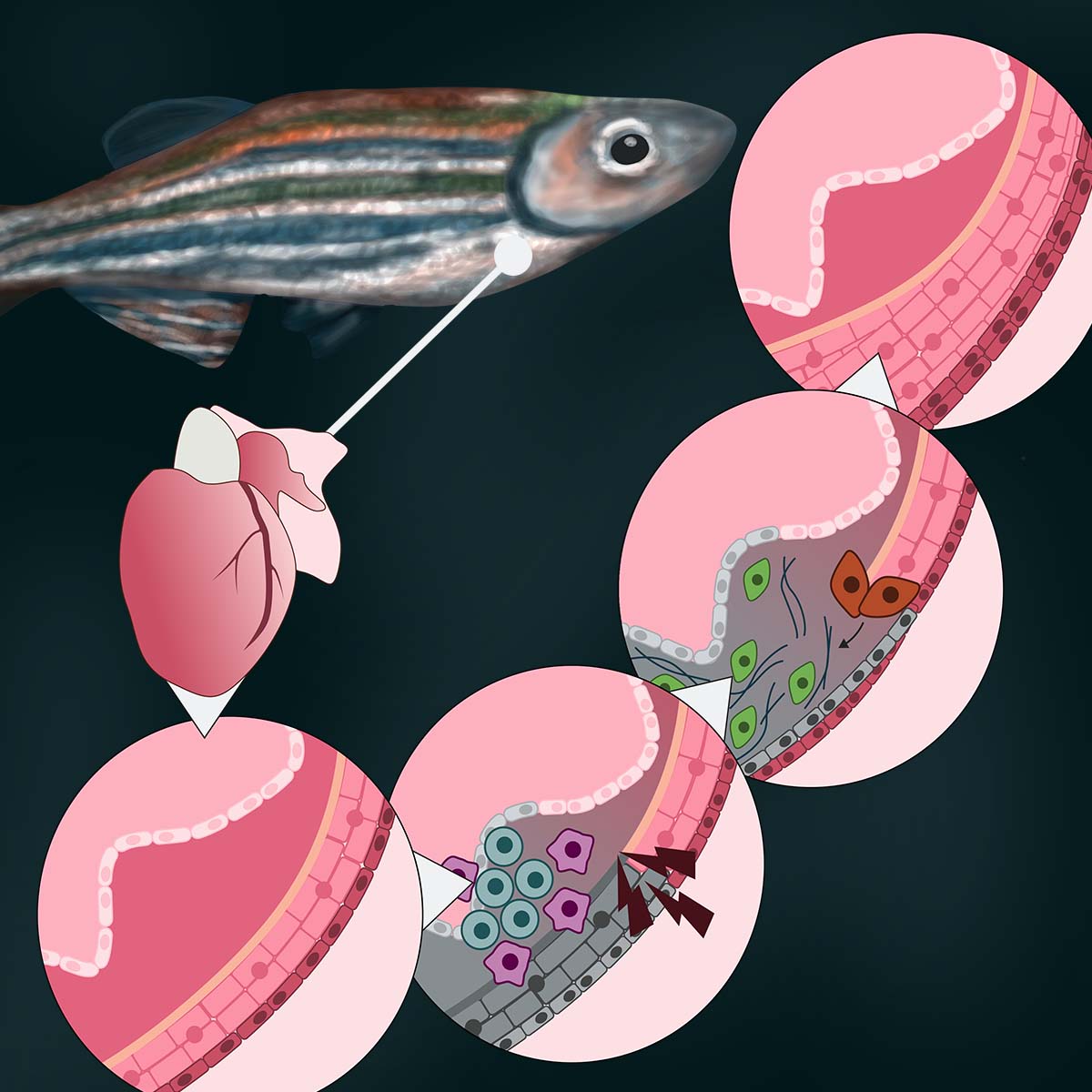
Ability to regenerate
Zebrafish have an astonishing ability to regenerate parts of their body, including heart muscle and even spinal cord tissue.
Dr. Haesemeyer is interested in thermoregulation, or the means by which animals maintain an optimal body temperature. Humans rely on involuntary processes such as sweating or constriction of blood vessels, but we also can use behavior — fan ourselves, say, or put on a sweater.
Fish, including zebrafish, lack any internal means of thermoregulation, making them ideal for the study of thermoregulation via behavior. In Dr. Haesemeyer’s words, “How does the animal find its comfortable temperature? How does it know which direction to swim?”
Basic research key to scientific progress
Better understanding that function, Dr. Haesemeyer explains, will help us understand how brains control things. Figuring out how thermoregulation works is important, he says, because when it goes awry, “There are very adverse consequences.”
“Knowing how it works in a healthy situation could help with fixing it when it’s broken,” he says.
It’s a common theme in the basic research done with the zebrafish model: Deciphering and describing a healthy system provides clues to managing an unhealthy one. “Diagnosing what’s wrong is so much easier if you know what ‘right’ is supposed to look like. It’s my pet peeve [that] so often in science we go after the disease first,” Dr. Haesemeyer says.
James Jontes, PhD , another zebrafish principal investigator who shares a facility with Dr. Haesemeyer, agrees.
Dr. Jontes, an associate professor in the Department of Neuroscience at the College of Medicine, studies cell adhesion molecules, a family of molecules that cause cells to stick together. He uses timelapse imaging to study the formation of neural networks in the brain.
“We can watch everything happen from the very first cell division, 10 minutes after [zebrafish embryos] are fertilized,” he says. “In two days, they’re swimming in short bursts; by five days, they’re hunting little microorganisms.”
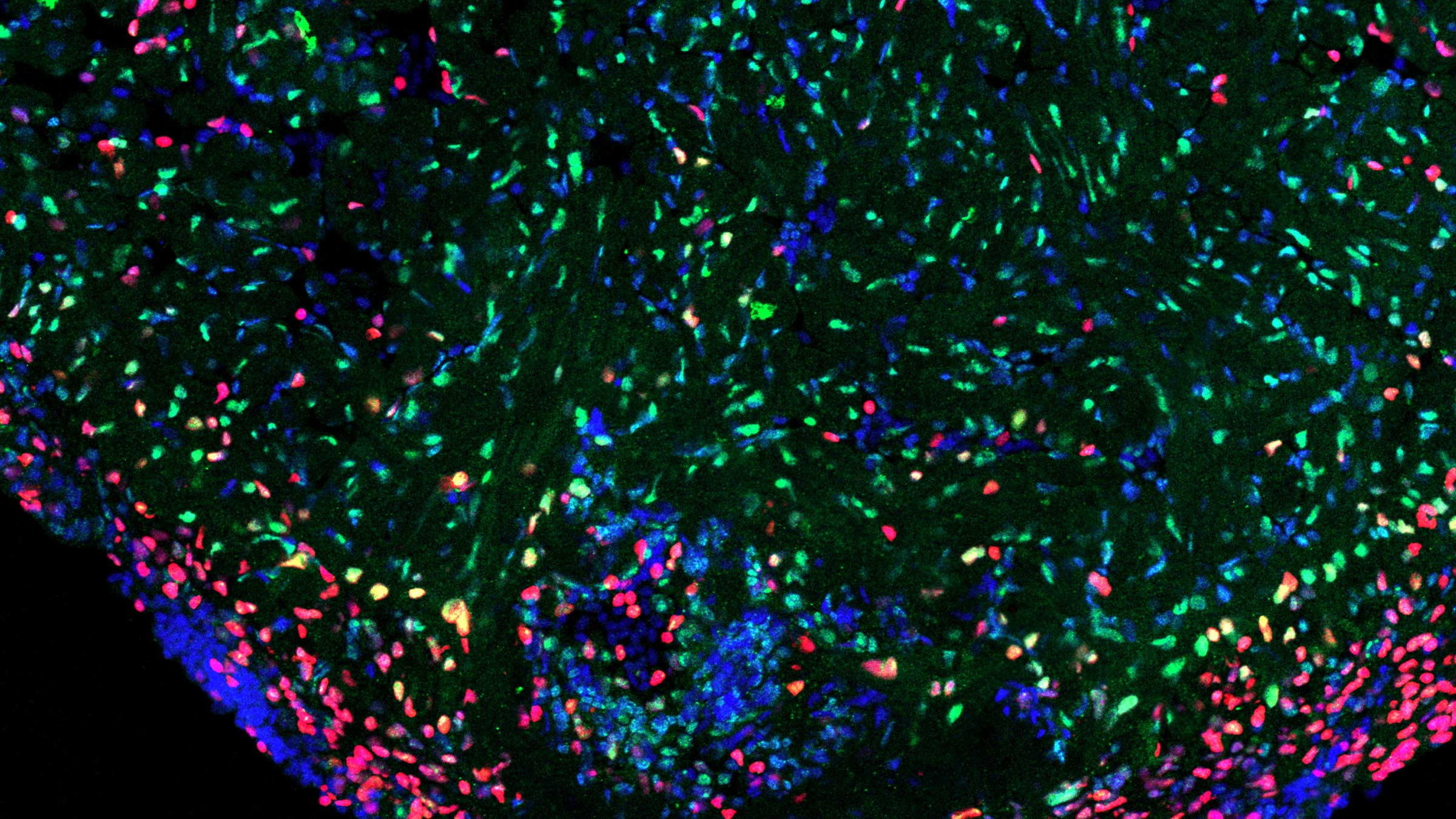
Aaron Goldman, PhD , an assistant professor in the College of Medicine Department of Biological Chemistry and Pharmacology, focuses his research on the zebrafish’s “profound ability to regenerate damaged heart muscle.” His goal is to learn more about what distinguishes this remarkable ability in zebrafish from the scarring and muscle dysfunction that occur in humans.
Ohio State’s newest zebrafish researcher, Lihua Ye, PhD , is in the second year of a five-year career development award from the National Institute of Diabetes and Digestive and Kidney Diseases. She’s an assistant professor in the Department of Neuroscience at the College of Medicine, and for her, zebrafish offer an ideal window on the gut-brain axis. That’s a two-way system of communication between the brain and the part of the nervous system that regulates immune and endocrine functions. This web of neurons is embedded in the wall of the gastrointestinal system from the esophagus to the rectum.
With a zebrafish embryo under a microscope, Dr. Ye can introduce bacteria to the gut and see, in real time, the brain’s response. She sees potential applications for her work such as discovering how the human body interprets and responds to what a person consumes and how gut bacteria regulate our food choices.
Dr. Ye’s work benefits from yet another zebrafish attribute: their ease of breeding.
“The fact that we can very easily generate germ-free fish and manipulate the composition of the zebrafish gut microbiome is very powerful,” she says.
She shares a fish facility with Bradley Blaser MD, PhD , a member of the Leukemia Research Program at The Ohio State University Comprehensive Cancer Center — Arthur G. James Cancer Hospital and Richard J. Solove Research Institute and assistant professor of hematology at the College of Medicine. Dr. Blaser’s lab examines how blood stem cells respond in development under normal conditions and under induced stress. The work could explain how changes in the genetic makeup of a person’s blood over a lifetime may contribute to certain blood cancers.
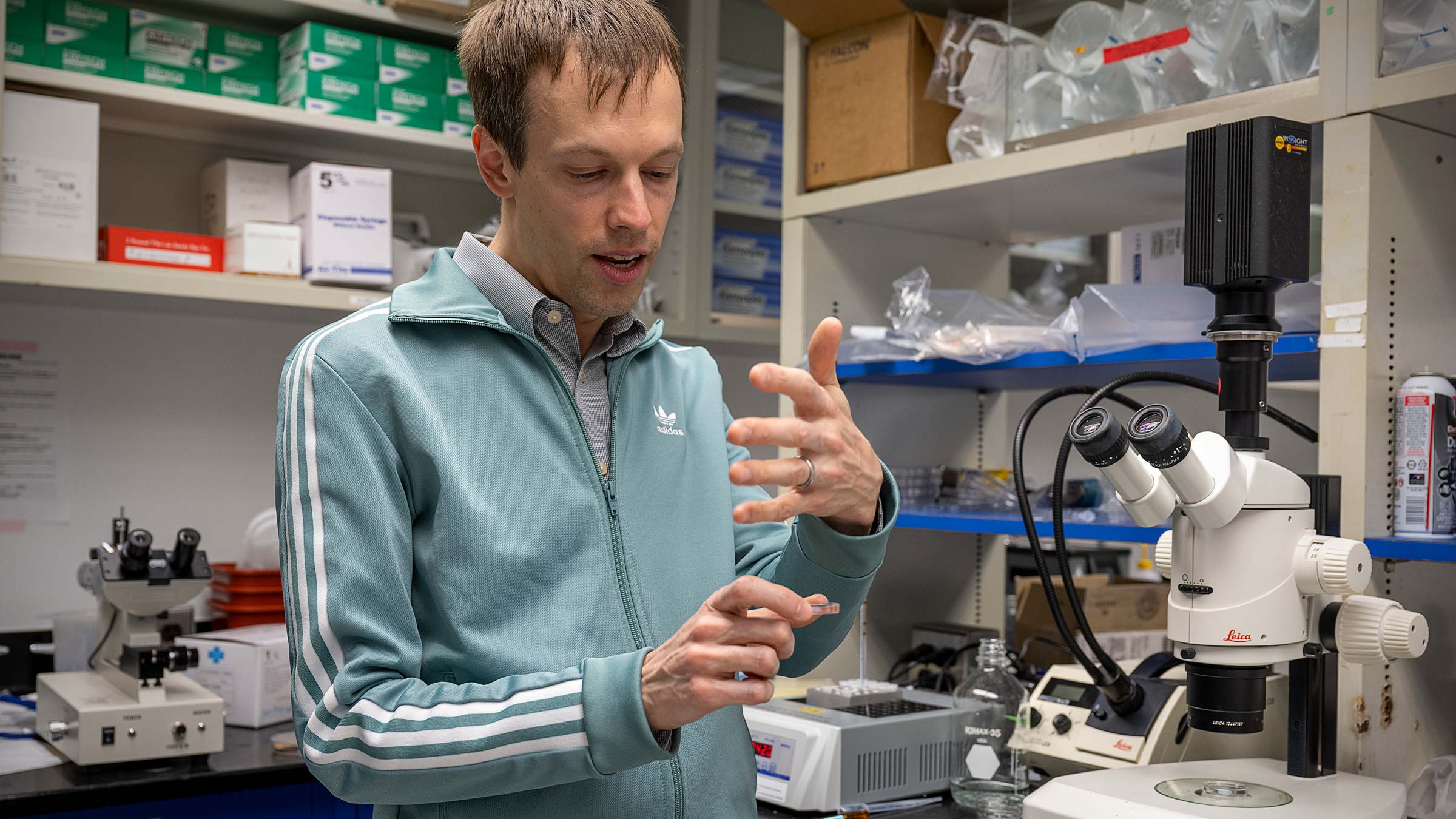
A seventh Ohio State-affiliated researcher using the zebrafish model is Genevieve Kendall, PhD , who has her lab at Nationwide Children’s Hospital in the Center for Childhood Cancer and also is an assistant professor of pediatrics in the College of Medicine. Her work focuses on the relationship between certain childhood cancers and a misregulation in the development of skeletal muscle.
Science moves forward on the work of many minds
Laboratory researchers like Ohio State’s zebrafish scientists expect that their findings will eventually be applied to solving clinical problems.
“Translational value is always the goal,” Dr. Ye says. But they know that world-changing breakthroughs typically are built on many years of the basic research that they and hundreds of other scientists are doing at the College of Medicine.
Dr. Jontes understands that people affected by a disease urgently desire a cure. But he argues we wouldn’t learn as much if we were just simply focused through the lens of a specific disease. An institution such as Ohio State, with dozens of labs asking a diverse array of questions, is primed for discovery because the value in science is having “a lot of people out there doing a lot of different things.”
“Ninety-nine percent of the time, that’s how things work,” he says. “Collectively, we make progress.”
When you give to The Ohio State University Wexner Medical Center, you’re helping improve lives
We’re committed to making advancements in research, education and patient care that will have an impact throughout Ohio and the world.
- Neurology ,
- Neuroscience ,
- Ohio State Wexner Medical Center ,
- OSUCCC – James
- Discovery & Innovation
- Research Advances
Related websites
- Ohio State's Comprehensive Cancer Center – James Cancer Hospital and Solove Research Institute
Articles on Discovery & Innovation
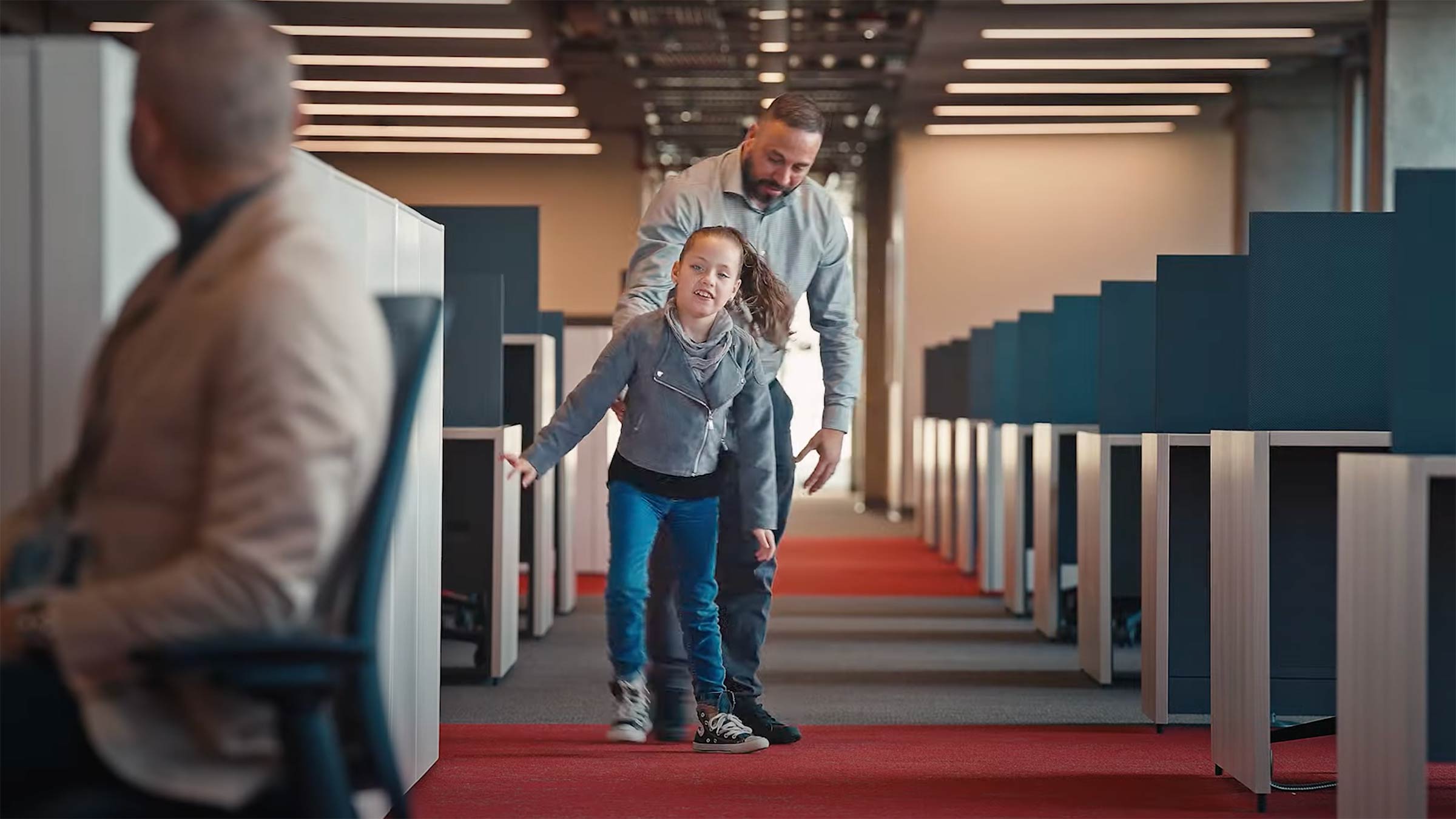
How gene therapy changed everything for child born with AADC deficiency
Ohio State physician-scientists developed a way to deliver gene therapy for children with a rare genetic disorder, leading to dramatic improvements in their ability to make even the most basic movements.
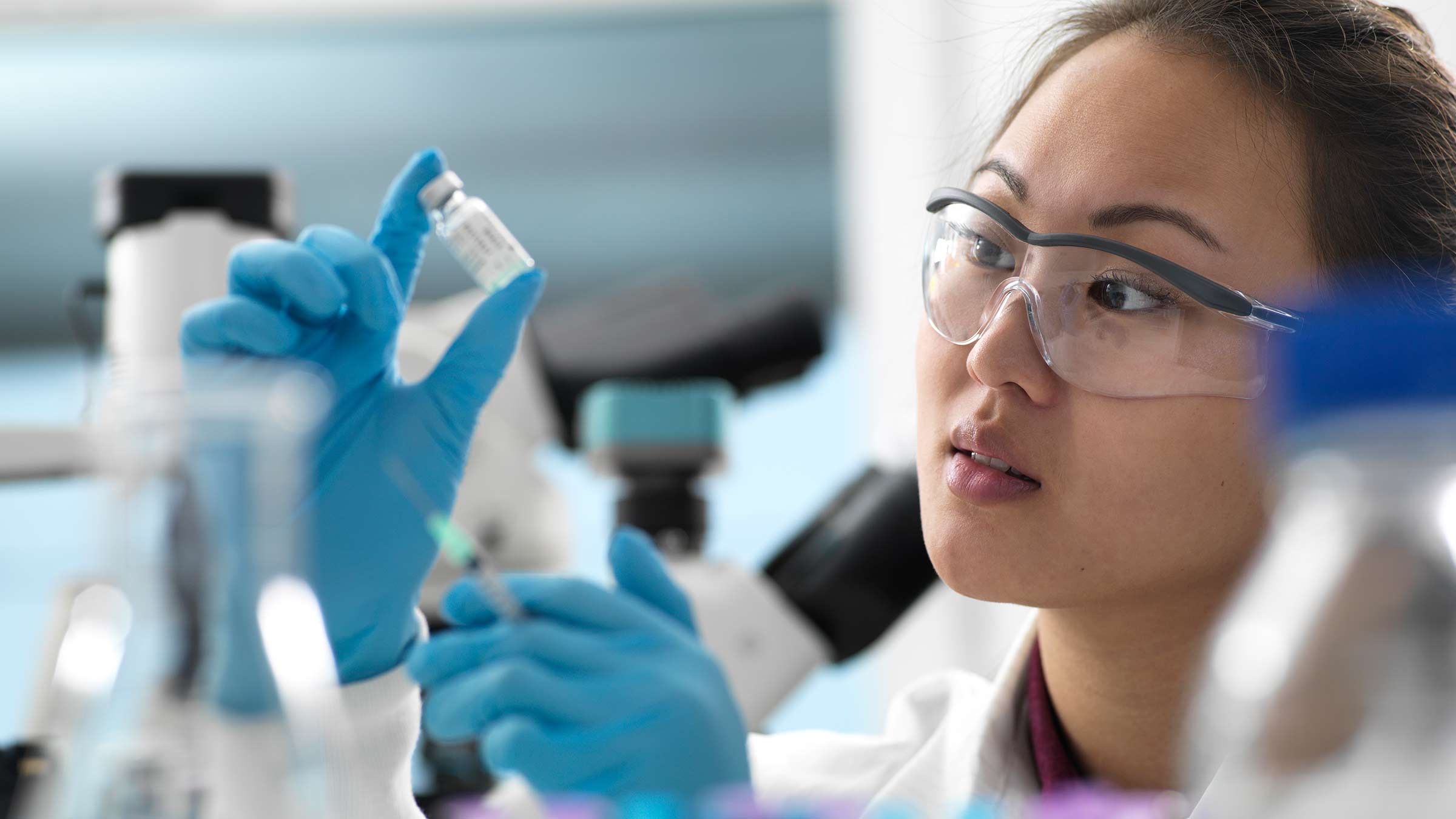
Why join a clinical trial?
By John L Hays, MD, PhD
Clinical trials make new medications and treatments available. Find out how clinical trials work from an Ohio State cancer researcher.
New ultra-high-frequency ultrasound improves lymphedema treatment
By Roman Skoracki, MD
Studying the intersection of obesity and pancreatic cancer to unlock early warning signs
By Wendy Margolin
How clinical trials work
By Julie Johnson, PharmD
Get articles and stories about health, wellness, medicine, science and education delivered right to your inbox from the experts at Ohio State.
Required fields
Tell us more about yourself
By clicking "Subscribe" you agree to our Terms of Use . Learn more about how we use your information by reading our Privacy Policy .
- Help & FAQ
Identification and analysis of genes involved in bone formation – a genetic approach in zebrafish –
- Hubrecht Institute
Research output : PhD Thesis › PhD thesis
T1 - Identification and analysis of genes involved in bone formation – a genetic approach in zebrafish –
AU - Spoorendonk, K.M.
N1 - Reporting year: 2009 Metis note: The full text of this item will be available after 17-12-2013
N2 - For many years bone research has been mainly performed in mice, chicken, cell culture systems, or human material from the clinic. In this thesis, we make use of the zebrafish (Danio rerio), a relatively new model system in this field. This small teleost offers possibilities which makes it a great complement to the mouse: forward genetic screens are possible in fish due to extra-uterine development and large brood size, and the recent generation of osteoblast-specific reporter lines allows visualization of osteoblasts in vivo. As key regulators of bone formation are highly conserved between mammals and teleosts, findings in fish likely apply to mammalian osteogenesis and tissue mineralization. Out of a forward genetic screen we performed, we identified 27 specific bone mutants which can be categorized in three different groups: (1) less or no bone, (2) ectopic over-ossification, and (3) more perichondral bone, but normal dermal bone. Two mutants out of the second class were analyzed in detail. The stocksteif mutant is characterized by over-ossification of the notochord resulting in fused vertebrae. stocksteif encodes cyp26b1, a retinoic acid metabolizing enzyme. We show that cyp26b1 is expressed in osteoblasts and that -upon retinoic acid treatment- osteoblasts enhance their activity. Consequently, the over-production of bone causes the fusion of the vertebrae. Thus, regulation of retinoic acid levels and the tight control of cyp26b1 activity are essential for regulation of skeletogenesis in zebrafish. The second mutant, dragonfish, is also characterized by over-ossification of the notochord and the subsequent fusion of the vertebrae. However, dragonfish functions in a pathway which is clearly distinct of that of retinoic acid. Additionally, ectopic mineralizations in the brain and neural tube can be observed in these mutants and, interestingly, at these spots the typical osteoblast-specific genes are not detected. Future research has to unravel the molecular lesion in these mutants and will provide novel insight into the regulation of bone development and disease.
AB - For many years bone research has been mainly performed in mice, chicken, cell culture systems, or human material from the clinic. In this thesis, we make use of the zebrafish (Danio rerio), a relatively new model system in this field. This small teleost offers possibilities which makes it a great complement to the mouse: forward genetic screens are possible in fish due to extra-uterine development and large brood size, and the recent generation of osteoblast-specific reporter lines allows visualization of osteoblasts in vivo. As key regulators of bone formation are highly conserved between mammals and teleosts, findings in fish likely apply to mammalian osteogenesis and tissue mineralization. Out of a forward genetic screen we performed, we identified 27 specific bone mutants which can be categorized in three different groups: (1) less or no bone, (2) ectopic over-ossification, and (3) more perichondral bone, but normal dermal bone. Two mutants out of the second class were analyzed in detail. The stocksteif mutant is characterized by over-ossification of the notochord resulting in fused vertebrae. stocksteif encodes cyp26b1, a retinoic acid metabolizing enzyme. We show that cyp26b1 is expressed in osteoblasts and that -upon retinoic acid treatment- osteoblasts enhance their activity. Consequently, the over-production of bone causes the fusion of the vertebrae. Thus, regulation of retinoic acid levels and the tight control of cyp26b1 activity are essential for regulation of skeletogenesis in zebrafish. The second mutant, dragonfish, is also characterized by over-ossification of the notochord and the subsequent fusion of the vertebrae. However, dragonfish functions in a pathway which is clearly distinct of that of retinoic acid. Additionally, ectopic mineralizations in the brain and neural tube can be observed in these mutants and, interestingly, at these spots the typical osteoblast-specific genes are not detected. Future research has to unravel the molecular lesion in these mutants and will provide novel insight into the regulation of bone development and disease.
M3 - PhD thesis (Proefschrift)
PB - Utrecht University
CY - Utrecht
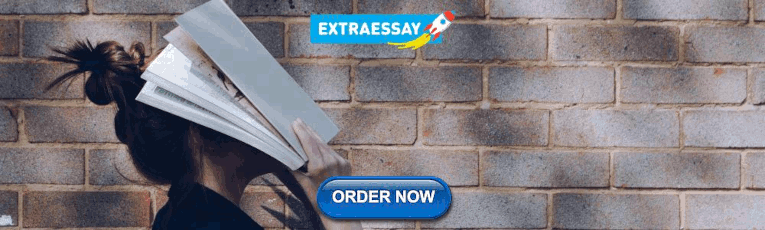
IMAGES
VIDEO
COMMENTS
A Thesis Submitted for the Degree of PhD . at the . University of St Andrews . 2018 . Full metadata for this thesis is available in ... 1.3.1 Zebrafish as a model to study neurodevelopmental disorders 35 1.3.2 Tools to study disorders in zebrafish 37 1.4 Summary and aims 45.
Mat and Mike for all the guidance and help with the zebrafish work throughout my PhD. I would also like to thank all the Firth court porters, Paul1, Paul2, Alan, Stuart and Steve for ... my thesis writing significantly more fun (p<0.001) compared to youtube. My deepest gratitude to mum, dad and brother, for their constant faith in me and being ...
ZEBRAFISH (DANIO RERIO) by Thomas H. Miller June, 2017 Director of Thesis: Dr. Fadi A. Issa, Ph.D. Major Department: Biology In zebrafish (Danio rerio), social interactions between adult males consist of a series of aggressive encounters that ultimately lead to the formation of stable hierarchies of either socially
zebrafish genetic models Yang Dong (1661360) Supervisor: Michael Lardelli Co-supervisor: Morgan Newman Department of Genetics and Evolution School of Biological Sciences A thesis for the degree of Doctor of Philosophy The University of Adelaide Submission Date: 14 October 2020
Dissertation Advisor: Florian Engert Author: Xiuye Chen iii . Dissection of complex behavior and whole-brain functional mapping in larval zebrafish . ABSTRACT. Understanding how the brain transforms sensory input into complex behavior is a fundamental question in systems neuroscience. Using larval zebrafish, we study the
In this dissertation, I present my efforts to develop the zebrafish as a model for neurodevelopmental disorders characterized by social impairments. I review the existing literature on early social development in zebrafish, characterize visually driven social behavior by developing a novel high-throughput assay, identify a region of the forebrain
Zebrafish as a translational model of Parkinson's disease - a study of microRNAs Lisa Trollope Thesis submitted for the degree of Doctor of Philosophy The University of Sheffield Faculty of Medicine, Dentistry and Health ... PhD process, allowing me freedom to develop as a scientist while ensuring I stay focused.
Zebrafish shoaling behavior: its development, quantification, ... A long-term endeavor such as PhD studies is never quite undertaken alone. I owe thanks to those who have supported me, scientifically, professionally, and personally. This thesis is a culmination of a long term working relationship with my advisor, Dr. Robert Gerlai. As a second ...
Zebrafish are a vertebrate model organism well suited for high throughput drug screens, and genome editing can be used to create heritable mutations in causative genes to model human disease. This thesis presents five genetic models of Parkinson's disease in the zebrafish created by CRISPR/Cas9 targeting (pink1, parkin, dj-1, fbxo7, and gba).
Michael Martin's contributions to the Thesis Aim 1: Development of tools for the dissection of neural circuits in the larval zebrafish. Michael Martin developed a pipeline for analysis of neural circuits in the larval zebrafish through development of hardware configurations, software and protocols, as well as adaptation and
1. Introduction. Zebrafish (Danio rerio) are tropical fish native to southern Asia and became a vertebrate model organism in developmental biology pioneered by George Streisinger in the 1970s at the University of Oregon.Since then, zebrafish have emerged as one of the animal models in preclinical studies for understanding various physiological processes and diseases.
Zebrafish are group spawners and egg scatterers, although females are choosy with respect to sites for oviposition and males defend territories around such sites. Laboratory studies of zebrafish behaviour have encompassed shoaling, foraging, reproduction, sensory perception and learning. These studies are reviewed in relation to the suitability ...
thesis examined sub-lethal adverse reproductive effects of chronic direct exposure of CBZ and GEM to F 0 zebrafish and several generations of unexposed offspring; the effects of exposure on testicular steroidogenesis were also examined. Chronic exposure of zebrafish to CBZ and GEM reduced ex vivo production of 11KT in testes.
Microbiome manipulations in zebrafish. The earliest zebrafish microbiome studies focused on the larval stage of zebrafish development 7, 8, which parallels the neonatal period of mammalian ...
Inhibitors via Automated Methods Using Zebrafish and the Discovery and Validation of PD 81723 as a Novel Angiogenesis Inhibitor Antonio N. Mauro Doctor of Philosophy Institute of Medical Science University of Toronto 2021 Abstract Their optical clarity as larvae and embryos, small size, and high fecundity make zebrafish ideal
THESIS THE ROLE OF FLNC IN THE CONTRACTILITY OF THE HEART AND VALVE DEVELOPMENT IN ZEBRAFISH Submitted by Areej Ali Alshahrani Department Of Biology In partial fulfillment of the requirements For the Degree of Master of Science Colorado State University Fort Collins, Colorado Spring 2020 Master's Committee: Advisor: Deborah Garrity
This chapter contains sections titled: Importance of Assessing Developmental Toxicity. Current Methods for Assessing Developmental Toxicity. Use of Emerging Models for Developmental Toxicity Testing. New Guidelines for Chemical Testing Using Zebrafish. Conclusions.
This thesis addresses these questions by investigating the behaviour and morphology of zebrafish deriving from several wild populations in Nepal and Bangladesh. Mate choice trials in the laboratory revealed several features of the zebrafish that make it less amenable to such studies than several alternative teleost species.
Lihua Ye, PhD, uses zebrafish in her study of the gut microbiome. Because zebrafish can be bred to be 'germ-free,' Dr. Ye can introduce controlled bacteria into the gut's microbiome to study the response. Those tankmates coexist by the thousands in three separate facilities on the medical center campus. Each is a sort of living genetic library ...
In this thesis, we make use of the zebrafish (Danio rerio), a relatively new model system in this field. This small teleost offers possibilities which makes it a great complement to the mouse: forward genetic screens are possible in fish due to extra-uterine development and large brood size, and the recent generation of osteoblast-specific ...
Groneberg's doctoral thesis explored how the motions of groups of zebrafish larvae affected each individual animal's brain development and behavior, teasing out the impact by raising some larvae in isolation and documenting any differences. "We used to joke that my Ph.D. was easily danceable as it's about movement," Groneberg says.
These sorptive losses decrease the concentration of chemical available to the zebrafish embryos, and therefore impact the interpretation of dose-response toxicity data. In an attempt to create a predictive model for sorptive losses, the measured sorption was modeled against the log K[subscript ow], molecular weight, and subcooled liquid ...
December 18, 2023. Ola earned her Master's degree in Neurosciences from Vrije Universiteit Amsterdam and conducted research for her master's thesis at Brigham and Women's Hospital at Harvard Medical School. There, she focused on developing patient-specific stem cell models to assess the safety and efficacy of antisense oligonucleotide therapy ...