- Open access
- Published: 19 September 2018
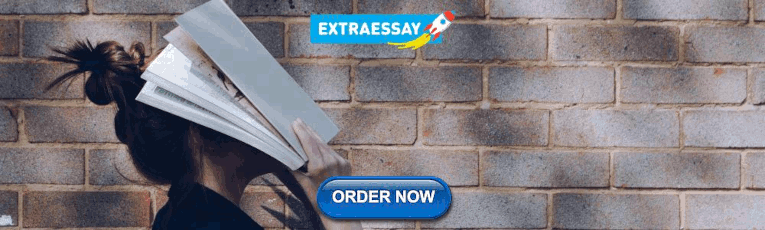
Nano based drug delivery systems: recent developments and future prospects
- Jayanta Kumar Patra ORCID: orcid.org/0000-0003-4118-4355 1 ,
- Gitishree Das 1 ,
- Leonardo Fernandes Fraceto 2 , 3 ,
- Estefania Vangelie Ramos Campos 2 , 3 ,
- Maria del Pilar Rodriguez-Torres ORCID: orcid.org/0000-0001-9107-247X 4 ,
- Laura Susana Acosta-Torres ORCID: orcid.org/0000-0002-5959-9113 4 ,
- Luis Armando Diaz-Torres ORCID: orcid.org/0000-0002-1281-9916 5 ,
- Renato Grillo 6 ,
- Mallappa Kumara Swamy 7 ,
- Shivesh Sharma 8 ,
- Solomon Habtemariam 9 &
- Han-Seung Shin 10
Journal of Nanobiotechnology volume 16 , Article number: 71 ( 2018 ) Cite this article
458k Accesses
3695 Citations
65 Altmetric
Metrics details
Nanomedicine and nano delivery systems are a relatively new but rapidly developing science where materials in the nanoscale range are employed to serve as means of diagnostic tools or to deliver therapeutic agents to specific targeted sites in a controlled manner. Nanotechnology offers multiple benefits in treating chronic human diseases by site-specific, and target-oriented delivery of precise medicines. Recently, there are a number of outstanding applications of the nanomedicine (chemotherapeutic agents, biological agents, immunotherapeutic agents etc.) in the treatment of various diseases. The current review, presents an updated summary of recent advances in the field of nanomedicines and nano based drug delivery systems through comprehensive scrutiny of the discovery and application of nanomaterials in improving both the efficacy of novel and old drugs (e.g., natural products) and selective diagnosis through disease marker molecules. The opportunities and challenges of nanomedicines in drug delivery from synthetic/natural sources to their clinical applications are also discussed. In addition, we have included information regarding the trends and perspectives in nanomedicine area.
Since ancient times, humans have widely used plant-based natural products as medicines against various diseases. Modern medicines are mainly derived from herbs on the basis of traditional knowledge and practices. Nearly, 25% of the major pharmaceutical compounds and their derivatives available today are obtained from natural resources [ 1 , 2 ]. Natural compounds with different molecular backgrounds present a basis for the discovery of novel drugs. A recent trend in the natural product-based drug discovery has been the interest in designing synthetically amenable lead molecules, which mimic their counterpart’s chemistry [ 3 ]. Natural products exhibit remarkable characteristics such as extraordinary chemical diversity, chemical and biological properties with macromolecular specificity and less toxicity. These make them favorable leads in the discovery of novel drugs [ 4 ]. Further, computational studies have helped envisage molecular interactions of drugs and develop next-generation drug inventions such as target-based drug discovery and drug delivery.
Despite several advantages, pharmaceutical companies are hesitant to invest more in natural product-based drug discovery and drug delivery systems [ 5 ] and instead explore the available chemical compounds libraries to discover novel drugs. However, natural compounds are now being screened for treating several major diseases, including cancer, diabetes, cardiovascular, inflammatory, and microbial diseases. This is mainly because natural drugs possess unique advantages, such as lower toxicity and side effects, low-price, and good therapeutic potential. However, concerns associated with the biocompatibility, and toxicity of natural compounds presents a greater challenge of using them as medicine. Consequently, many natural compounds are not clearing the clinical trial phases because of these problems [ 6 , 7 , 8 ]. The use of large sized materials in drug delivery poses major challenges, including in vivo instability, poor bioavailability, and poor solubility, poor absorption in the body, issues with target-specific delivery, and tonic effectiveness, and probable adverse effects of drugs. Therefore, using new drug delivery systems for targeting drugs to specific body parts could be an option that might solve these critical issues [ 9 , 10 ]. Hence, nanotechnology plays a significant role in advanced medicine/drug formulations, targeting arena and their controlled drug release and delivery with immense success.
Nanotechnology is shown to bridge the barrier of biological and physical sciences by applying nanostructures and nanophases at various fields of science [ 11 ]; specially in nanomedicine and nano based drug delivery systems, where such particles are of major interest [ 12 , 13 ]. Nanomaterials can be well-defined as a material with sizes ranged between 1 and 100 nm, which influences the frontiers of nanomedicine starting from biosensors, microfluidics, drug delivery, and microarray tests to tissue engineering [ 14 , 15 , 16 ]. Nanotechnology employs curative agents at the nanoscale level to develop nanomedicines. The field of biomedicine comprising nanobiotechnology, drug delivery, biosensors, and tissue engineering has been powered by nanoparticles [ 17 ]. As nanoparticles comprise materials designed at the atomic or molecular level, they are usually small sized nanospheres [ 18 ]. Hence, they can move more freely in the human body as compared to bigger materials. Nanoscale sized particles exhibit unique structural, chemical, mechanical, magnetic, electrical, and biological properties. Nanomedicines have become well appreciated in recent times due to the fact that nanostructures could be utilized as delivery agents by encapsulating drugs or attaching therapeutic drugs and deliver them to target tissues more precisely with a controlled release [ 10 , 19 ]. Nanomedicine, is an emerging field implementing the use of knowledge and techniques of nanoscience in medical biology and disease prevention and remediation. It implicates the utilization of nanodimensional materials including nanorobots, nanosensors for diagnosis, delivery, and sensory purposes, and actuate materials in live cells (Fig. 1 ). For example, a nanoparticle-based method has been developed which combined both the treatment and imaging modalities of cancer diagnosis [ 20 ]. The very first generation of nanoparticle-based therapy included lipid systems like liposomes and micelles, which are now FDA-approved [ 21 ]. These liposomes and micelles can contain inorganic nanoparticles like gold or magnetic nanoparticles [ 22 ]. These properties let to an increase in the use of inorganic nanoparticles with an emphasis on drug delivery, imaging and therapeutics functions. In addition, nanostructures reportedly aid in preventing drugs from being tarnished in the gastrointestinal region and help the delivery of sparingly water-soluble drugs to their target location. Nanodrugs show higher oral bioavailability because they exhibit typical uptake mechanisms of absorptive endocytosis.
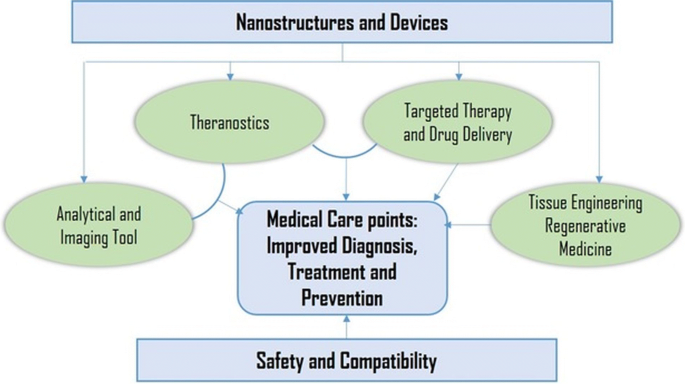
Application and goals of nanomedicine in different sphere of biomedical research
Nanostructures stay in the blood circulatory system for a prolonged period and enable the release of amalgamated drugs as per the specified dose. Thus, they cause fewer plasma fluctuations with reduced adverse effects [ 23 ]. Being nanosized, these structures penetrate in the tissue system, facilitate easy uptake of the drug by cells, permit an efficient drug delivery, and ensure action at the targeted location. The uptake of nanostructures by cells is much higher than that of large particles with size ranging between 1 and 10 µm [ 17 , 24 ]. Hence, they directly interact to treat the diseased cells with improved efficiency and reduced or negligible side effects.
At all stages of clinical practices, nanoparticles have been found to be useful in acquiring information owing to their use in numerous novel assays to treat and diagnose diseases. The main benefits of these nanoparticles are associated with their surface properties; as various proteins can be affixed to the surface. For instance, gold nanoparticles are used as biomarkers and tumor labels for various biomolecule detection procedural assays.
Regarding the use of nanomaterials in drug delivery, the selection of the nanoparticle is based on the physicochemical features of drugs. The combined use of nanoscience along with bioactive natural compounds is very attractive, and growing very rapidly in recent times. It presents several advantages when it comes to the delivery of natural products for treating cancer and many other diseases. Natural compounds have been comprehensively studied in curing diseases owing to their various characteristic activities, such as inducing tumor-suppressing autophagy and acting as antimicrobial agents. Autophagy has been observed in curcumin and caffeine [ 25 ], whereas antimicrobial effects have been shown by cinnamaldehyde, carvacrol, curcumin and eugenol [ 26 , 27 ]. The enrichment of their properties, such as bioavailability, targeting and controlled release were made by incorporating nanoparticles. For instance, thymoquinone, a bioactive compound in Nigella sativa , is studied after its encapsulation in lipid nanocarrier. After encapsulation, it showed sixfold increase in bioavailability in comparison to free thymoquinone and thus protects the gastrointestinal stuffs [ 28 ]. It also increased the pharmacokinetic characteristics of the natural product resulting in better therapeutic effects.
Metallic, organic, inorganic and polymeric nanostructures, including dendrimers, micelles, and liposomes are frequently considered in designing the target-specific drug delivery systems. In particular, those drugs having poor solubility with less absorption ability are tagged with these nanoparticles [ 17 , 29 ]. However, the efficacy of these nanostructures as drug delivery vehicles varies depending on the size, shape, and other inherent biophysical/chemical characteristics. For instance, polymeric nanomaterials with diameters ranging from 10 to 1000 nm, exhibit characteristics ideal for an efficient delivery vehicle [ 7 ]. Because of their high biocompatibility and biodegradability properties, various synthetic polymers such as polyvinyl alcohol, poly- l -lactic acid, polyethylene glycol, and poly(lactic- co -glycolic acid), and natural polymers, such as alginate and chitosan, are extensively used in the nanofabrication of nanoparticles [ 8 , 30 , 31 , 32 ]. Polymeric nanoparticles can be categorized into nanospheres and nanocapsules both of which are excellent drug delivery systems. Likewise, compact lipid nanostructures and phospholipids including liposomes and micelles are very useful in targeted drug delivery.
The use of ideal nano-drug delivery system is decided primarily based on the biophysical and biochemical properties of the targeted drugs being selected for the treatment [ 8 ]. However, problems such as toxicity exhibited by nanoparticles cannot be ignored when considering the use of nanomedicine. More recently, nanoparticles have mostly been used in combination with natural products to lower the toxicity issues. The green chemistry route of designing nanoparticles loaded with drugs is widely encouraged as it minimises the hazardous constituents in the biosynthetic process. Thus, using green nanoparticles for drug delivery can lessen the side-effects of the medications [ 19 ]. Moreover, adjustments in nanostructures size, shape, hydrophobicity, and surface changes can further enhance the bioactivity of these nanomaterials.
Thus, nanotechnology offers multiple benefits in treating chronic human diseases by site-specific, and target-oriented delivery of medicines. However, inadequate knowledge about nanostructures toxicity is a major worry and undoubtedly warrants further research to improve the efficacy with higher safety to enable safer practical implementation of these medicines. Therefore, cautiously designing these nanoparticles could be helpful in tackling the problems associated with their use. Considering the above facts, this review aims to report different nano based drug delivery systems, significant applications of natural compound-based nanomedicines, and bioavailability, targeting sites, and controlled release of nano-drugs, as well as other challenges associated with nanomaterials in medicines.
Nano based drug delivery systems
Recently, there has been enormous developments in the field of delivery systems to provide therapeutic agents or natural based active compounds to its target location for treatment of various aliments [ 33 , 34 ]. There are a number of drug delivery systems successfully employed in the recent times, however there are still certain challenges that need to be addresses and an advanced technology need to be developed for successful delivery of drugs to its target sites. Hence the nano based drug delivery systems are currently been studied that will facilitate the advanced system of drug delivery.
Fundamentals of nanotechnology based techniques in designing of drug
Nanomedicine is the branch of medicine that utilizes the science of nanotechnology in the preclusion and cure of various diseases using the nanoscale materials, such as biocompatible nanoparticles [ 35 ] and nanorobots [ 36 ], for various applications including, diagnosis [ 37 ], delivery [ 38 ], sensory [ 39 ], or actuation purposes in a living organism [ 40 ]. Drugs with very low solubility possess various biopharmaceutical delivery issues including limited bio accessibility after intake through mouth, less diffusion capacity into the outer membrane, require more quantity for intravenous intake and unwanted after-effects preceding traditional formulated vaccination process. However all these limitations could be overcome by the application of nanotechnology approaches in the drug delivery mechanism.
Drug designing at the nanoscale has been studied extensively and is by far, the most advanced technology in the area of nanoparticle applications because of its potential advantages such as the possibility to modify properties like solubility, drug release profiles, diffusivity, bioavailability and immunogenicity. This, can consequently lead to the improvement and development of convenient administration routes, lower toxicity, fewer side effects, improved biodistribution and extended drug life cycle [ 17 ]. The engineered drug delivery systems are either targeted to a particular location or are intended for the controlled release of therapeutic agents at a particular site. Their formation involves self-assembly where in well-defined structures or patterns spontaneously are formed from building blocks [ 41 ]. Additionally they need to overcome barriers like opsonization/sequestration by the mononuclear phagocyte system [ 42 ].
There are two ways through which nanostructures deliver drugs: passive and self-delivery. In the former, drugs are incorporated in the inner cavity of the structure mainly via the hydrophobic effect. When the nanostructure materials are targeted to a particular sites, the intended amount of the drug is released because of the low content of the drugs which is encapsulated in a hydrophobic environment [ 41 ]. Conversely, in the latter, the drugs intended for release are directly conjugated to the carrier nanostructure material for easy delivery. In this approach, the timing of release is crucial as the drug will not reach the target site and it dissociates from the carrier very quickly, and conversely, its bioactivity and efficacy will be decreased if it is released from its nanocarrier system at the right time [ 41 ]. Targeting of drugs is another significant aspect that uses nanomaterials or nanoformulations as the drug delivery systems and, is classified into active and passive. In active targeting, moieties, such as antibodies and peptides are coupled with drug delivery system to anchor them to the receptor structures expressed at the target site. In passive targeting, the prepared drug carrier complex circulates through the bloodstream and is driven to the target site by affinity or binding influenced by properties like pH, temperature, molecular site and shape. The main targets in the body are the receptors on cell membranes, lipid components of the cell membrane and antigens or proteins on the cell surfaces [ 43 ]. Currently, most nanotechnology-mediated drug delivery system are targeted towards the cancer disease and its cure.
Biopolymeric nanoparticles in diagnosis, detection and imaging
The integration of therapy and diagnosis is defined as theranostic and is being extensively utilized for cancer treatment [ 44 , 45 ]. Theranostic nanoparticles can help diagnose the disease, report the location, identify the stage of the disease, and provide information about the treatment response. In addition, such nanoparticles can carry a therapeutic agent for the tumor, which can provide the necessary concentrations of the therapeutic agent via molecular and/or external stimuli [ 44 , 45 ]. Chitosan is a biopolymer which possesses distinctive properties with biocompatibility and presence of functional groups [ 45 , 46 , 47 ]. It is used in the encapsulation or coating of various types of nanoparticles, thus producing different particles with multiple functions for their potential uses in the detection and diagnosis of different types of diseases [ 45 , 47 ].
Lee et al. [ 48 ] encapsulated oleic acid-coated FeO nanoparticles in oleic acid-conjugated chitosan (oleyl-chitosan) to examine the accretion of these nanoparticles in tumor cells through the penetrability and holding (EPR) consequence under the in vivo state for analytical uses by the near-infrared and magnetic resonance imaging (MRI) mechanisms. By the in vivo evaluations, both techniques showed noticeable signal strength and improvement in the tumor tissues through a higher EPR consequence after the injection of cyanine-5-attached oleyl-chitosan nanoparticles intravenously (Cyanine 5).
Yang et al. [ 49 ] prepared highly effective nanoparticles for revealing colorectal cancer (CC) cells via a light-mediated mechanism; these cells are visible owing to the physical conjugation of alginate with folic acid-modified chitosan leading to the formation of nanoparticles with enhanced 5-aminolevulinic (5-ALA) release in the cell lysosome. The results displayed that the engineered nanoparticles were voluntarily endocytosed by the CC cells by the folate receptor-based endocytosis process. Subsequently, the charged 5-ALA was dispersed into the lysosome which was triggered by less desirability strength between the 5-ALA and chitosan through deprotonated alginate that gave rise to the gathering of protoporphyrin IX (PpIX) for photodynamic detection within the cells. As per this research, chitosan-based nanoparticles in combination with alginate and folic acid are tremendous vectors for the definite delivery of 5-ALA to the CC cells to enable endoscopic fluorescent detection. Cathepsin B (CB) is strongly associated with the metastatic process and is available in surplus in the pericellular areas where this process occurs; thus, CB is important for the detection of metastasis. Ryu et al. [ 50 ] designed a CB-sensitive nanoprobe (CB-CNP) comprising a self-satisfied CB-CNP with a fluorogenic peptide attached to the tumor-targeting glycol chitosan nanoparticles (CNPs) on its surface. The designed nanoprobe is a sphere with a diameter of 280 nm, with spherical structure and its fluorescence capacity was completely extinguished under the biological condition. The evaluation of the usability of CB-sensitive nanoprobe in three rat metastatic models demonstrated the potential of these nonoprobes in discriminating metastatic cells from healthy ones through non-invasive imaging. Hyaluronic acid (HA) is another biopolymeric material. This is a biocompatible, negatively charged glycosaminoglycan, and is one of the main constituents of the extracellular matrix [ 51 , 52 ]. HA can bind to the CD44 receptor, which is mostly over articulated in various cancerous cells, through the receptor-linker interaction. Thus, HA-modified nanoparticles are intriguing for their use in the detection and cure of cancer [ 53 , 54 , 55 ]. Wang et al. [ 56 ], coated the surface of iron oxide nanoparticles (IONP) with dopamine-modified HA. These nanoparticles have a hydrophilic exterior and a hydrophobic interior where the chemotherapeutic homocamptothecin is encapsulated [ 56 ]. The biopotential of this process was investigated in both laboratory and in the live cells. Increased uptake of nanoparticles by tumor cells was observed by MRI when an external magnetic field was employed [ 56 ]. After the intravenous administration of the nano-vehicle in 3 mg/kg (relative to the free drug) rats, a large tumor ablation was observed and after treatment, the tumors almost disappeared [ 56 ].
Choi et al. [ 53 ] also synthesized nanoparticles of hyaluronic acid with different diameters by changing the degree of hydrophobic replacement of HA. The nanoparticles were systemically administered in the mice with tumor, and then, its effect was studied. This same research group developed a versatile thermostatic system using poly (ethylene glycol) conjugated hyaluronic acid (P-HA-NPs) nanoparticles for the early detection of colon cancer and targeted therapy. To assess the effectiveness of the nanoparticles, they were first attached to the near-infrared fluorescent dye (Cy 5.5) by chemical conjugation, and then, the irinotecan anticancer drug (IRT) was encapsulated within these systems. The therapeutic potential of P-HA-NP was then investigated in different systems of the mice colon cancer. Through the intravenous injection of the fluorescent dye attached nanoparticles (Cy 5.5-P-HA-NPs), minute and initial-stage tumors as well as liver-embedded colon tumors were efficiently pictured using an NIRF imaging method. Due to their extraordinary capability to target tumors, drug-containing nanoparticles (IRT-P-HA-NP) showed markedly decreased tumor development with decreased systemic harmfulness. In addition, healing effects could be examined concurrently with Cy 5.5-P-HA-NPs [ 57 ].
Another option that can be used is alginate, which is a natural polymer derived from the brown seaweed and has been expansively scrutinized for its potential uses in the biomedical field because of its several favorable characteristics, such as low cost of manufacture, harmonious nature, less harmfulness, and easy gelling in response to the addition of divalent cations [ 58 , 59 ]. Baghbani et al. [ 60 ] prepared perfluorohexane (PFH) nanodroplets stabilized with alginate to drive doxorubicin and then evaluated their sensitivity to ultrasound and imaging as well as their therapeutic properties. Further found that the ultrasound-facilitated treatment with PFH nanodroplets loaded with doxorubicin exhibited promising positive responses in the breast cancer rat models. The efficacy was characterized by the deterioration of the tumor [ 60 ]. In another study, Podgorna et al. [ 61 ] prepared gadolinium (GdNG) containing nanogels for hydrophilic drug loading and to enable screening by MRI. The gadolinium alginate nanogels had an average diameter of 110 nm with stability duration of 60 days. Because of their paramagnetic behavior, the gadolinium mixtures are normally used as positive contrast agents (T1) in the MRI images. Gadolinium nanogels significantly reduce the relaxation time (T1) compared to controls. Therefore, alginate nanogels act as contrast-enhancing agents and can be assumed as an appropriate material for pharmacological application.
Also, the polymeric material dextran is a neutral polymer and is assumed as the first notable example of microbial exopolysaccharides used in medical applications. A remarkable advantage of using dextran is that it is well-tolerated, non-toxic, and biodegradable in humans, with no reactions in the body [ 62 ]. Photodynamic therapy is a site-specific cancer cure with less damage to non-cancerous cells. Ding et al. [ 63 ] prepared a nanoparticulate multifunctional composite system by encapsulating Fe 3 O 4 nanoparticles in dextran nanoparticles conjugated to redox-responsive chlorine 6 (C6) for near infrared (NIR) and magnetic resonance (MR) imaging. The nanoparticles exhibited an “off/on” behavior of the redox cellular response of the fluorescence signal, thus resulting in accurate imaging of the tumor. In addition, excellent in vitro and in vivo magnetic targeting ability was observed, contributing to the efficacy of enhanced photodynamic therapy. Hong et al. [ 64 ] prepared theranostic nanoparticles or glioma cells of C6 mice. These particles comprised of gadolinium oxide nanoparticles coated with folic acid-conjugated dextran (FA) or paclitaxel (PTX). The bioprotective effects of dextran coating and the chemotherapeutic effect of PTX on the C6 glioma cells were evaluated by the MTT assay. The synthesized nanoparticles have been shown to enter C6 tumor cells by receptor-mediated endocytosis and provide enhanced contrast (MR) concentration-dependent activity due to the paramagnetic property of the gadolinium nanoparticle. Multifunctional nanoparticles were more effective in reducing cell viability than uncoated gadolinium nanoparticles. Therefore, FA and PTX conjugated nanoparticles can be used as theranostic agents with paramagnetic and chemotherapeutic properties.
Drug designing and drug delivery process and mechanism
With the progression of nanomedicine and, due to the advancement of drug discovery/design and drug delivery systems, numerous therapeutic procedures have been proposed and traditional clinical diagnostic methods have been studied, to increase the drug specificity and diagnostic accuracy. For instance, new routes of drug administration are being explored, and there is focus on ensuring their targeted action in specific regions, thus reducing their toxicity and increasing their bioavailability in the organism [ 65 ].
In this context, drug designing has been a promising feature that characterizes the discovery of novel lead drugs based on the knowledge of a biological target. The advancements in computer sciences, and the progression of experimental procedures for the categorization and purification of proteins, peptides, and biological targets are essential for the growth and development of this sector [ 66 , 67 ]. In addition, several studies and reviews have been found in this area; they focus on the rational design of different molecules and show the importance of studying different mechanisms of drug release [ 68 ]. Moreover, natural products can provide feasible and interesting solutions to address the drug design challenges, and can serve as an inspiration for drug discovery with desired physicochemical properties [ 3 , 69 , 70 ].
Also, the drug delivery systems have been gaining importance in the last few years. Such systems can be easily developed and are capable of promoting the modified release of the active ingredients in the body. For example, Chen et al. [ 70 ] described an interesting review using nanocarriers for imaging and sensory applications and discussed the, therapy effect of these systems. In addition, Pelaz et al. [ 71 ] provided an up-to-date overview of several applications of nanocarriers to nanomedicine and discussed new opportunities and challenges for this sector.
Interestingly, each of these drug delivery systems has its own chemical, physical and morphological characteristics, and may have affinity for different drugs polarities through chemical interactions (e.g., covalent bonds and hydrogen bonds) or physical interactions (e.g., electrostatic and van der Waals interactions). As an example, Mattos et al. [ 72 ] demonstrated that, the release profile of neem bark extract-grafted biogenic silica nanoparticles (chemical interactions) was lower than neem bark extract-loaded biogenic silica nanoparticles. Hence, all these factors influence the interaction of nanocarriers with biological systems [ 73 ], as well as the release kinetics of the active ingredient in the organism [ 68 ]. In addition, Sethi et al. [ 74 ] designed a crosslinkable lipid shell (CLS) containing docetaxel and wortmannin as the prototypical drugs used for controlling the drug discharge kinetics; then, they studied, its discharge profile, which was found to be affected in both in vivo and in vitro conditions. Apart from this, other parameters, such as the composition of the nanocarriers (e.g., organic, inorganic, and hybrid materials) and the form in which drugs are associated with them (such as core–shell system or matrix system) are also fundamental for understanding their drug delivery profile [ 75 , 76 ]. Taken together, several studies regarding release mechanisms of drugs in nanocarriers have been conducted. Diffusion, solvent, chemical reaction, and stimuli-controlled release are a few mechanisms that can represent the release of drugs in nanocarriers as shown in Fig. 2 [ 77 , 78 ]. Kamaly et al. [ 79 ] provided a widespread review of controlled-release systems with a focus on studies related to controlling drug release from polymeric nanocarriers.
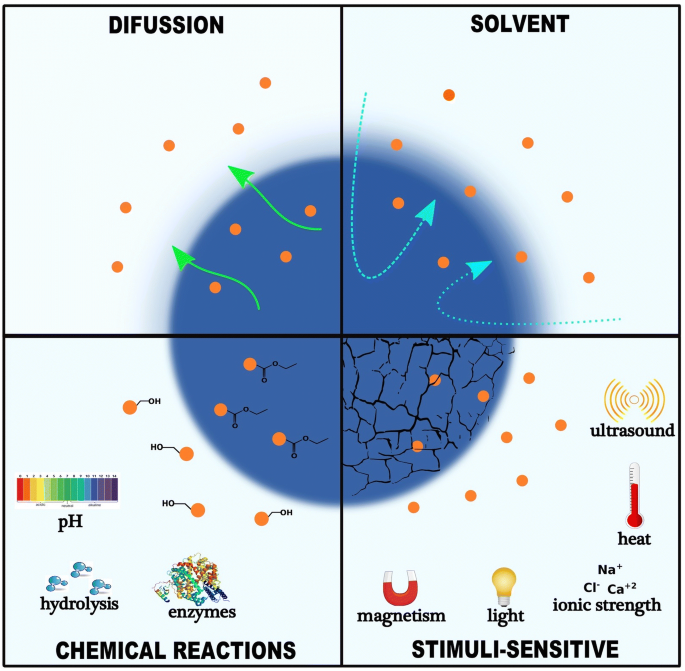
Mechanisms for controlled release of drugs using different types of nanocarriers
Although there are several nanocarriers with different drug release profiles, strategies are currently being formulated to improve the specificity of the nanostructures to target regions of the organism [ 80 ], and to reduce the immunogenicity through their coating or chemical functionalization with several substances, such as polymers [ 81 ], natural polysaccharides [ 82 , 83 ], antibodies [ 84 ], cell-membrane [ 85 ], and tunable surfactants [ 86 ], peptides [ 87 ], etc. In some cases where drugs do not display binding and affinity with a specific target or do not cross certain barriers (e.g. blood–brain barrier or the blood–cerebrospinal fluid barrier) [ 88 ], these ligand-modified nanocarriers have been used to pass through the cell membrane and allow a programmed drug delivery in a particular environment. For example, hyaluronic acid (a polysaccharide found in the extracellular matrix) has been used as a ligand-appended in several nanocarriers, showing promising results to boost antitumor action against the melanoma stem-like cells [ 89 ], breast cancer cells [ 90 ], pulmonary adenocarcinoma cells [ 91 ], as well as to facilitate intravitreal drug delivery for retinal gene therapy [ 83 ] and to reduce the immunogenicity of the formed protein corona [ 82 ]. However, the construction of the ligand-appended drug delivery systems is labor-intensive, and several targeting designs must be performed previously, taking into account the physiological variables of blood flow, disease status, and tissue architecture [ 92 ]. Moreover, few studies have been performed to evaluate the interaction of the ligand-appended in nanocarriers with cell membranes, and also their uptake mechanism is still unclear. Furthermore, has been known that the uptake of the nanoparticles by the cells occurs via phagocytic or non-phagocytic pathways (e.x. clathrin-mediated endocytosis, caveolae-mediated endocytosis, and others) [ 93 , 94 ], meanwhile due some particular physicochemical characteristics of each delivery systems have been difficult to standardize the mechanism of action/interaction of these systems in the cells. For example, Salatin and Khosroushahi [ 95 ], in a review highlighted the main endocytosis mechanisms responsible for the cellular uptake of polysaccharide nanoparticles containing active compounds.
On the other hand, stimuli-responsive nanocarriers have shown the ability to control the release profile of drugs (as a triggered release) using external factors such as ultrasound [ 96 ], heat [ 97 , 98 , 99 ], magnetism [ 100 , 101 ], light [ 102 ], pH [ 103 ], and ionic strength [ 104 ], which can improve the targeting and allow greater dosage control (Fig. 2 ). For example, superparamagnetic iron oxide nanoparticles are associated with polymeric nanocarriers [ 105 ] or lipids [ 106 ] to initially stimulate a controlled release system by the application of external magnetic field. In addition, Ulbrich et al. [ 107 ] revised recent achievements of drug delivery systems, in particular, on the basis of polymeric and magnetic nanoparticles, and also addressed the effect of covalently or noncovalently attached drugs for cancer cure [ 107 ]. Moreover, Au/Fe 3 O 4 @polymer nanoparticles have also been synthesized for the use in NIR-triggered chemo-photothermal therapy [ 108 ]. Therefore, hybrid nanocarriers are currently among the most promising tools for nanomedicine as they present a mixture of properties of different systems in a single system, thus ensuring materials with enhanced performance for both therapeutic and diagnostic applications (i.e., theranostic systems). Despite this, little is known about the real mechanisms of action and toxicity of drug delivery systems, which open opportunity for new studies. In addition, studies focusing on the synthesis of nanocarriers based on environmentally safe chemical reactions by implementing plant extracts and microorganisms have increased [ 10 ].
Nanoparticles used in drug delivery system
Biopolymeric nanoparticles.
There are numerous biopolymeric materials that are utilized in the drug delivery systems. These materials and their properties are discussed below.
Chitosan exhibits muco-adhesive properties and can be used to act in the tight epithelial junctions. Thus, chitosan-based nanomaterials are widely used for continued drug release systems for various types of epithelia, including buccal [ 109 ], intestinal [ 110 ], nasal [ 111 ], eye [ 112 ] and pulmonary [ 113 ]. Silva et al. [ 114 ] prepared and evaluated the efficacy of a 0.75% w/w isotonic solution of hydroxypropyl methylcellulose (HPMC) containing chitosan/sodium tripolyphosphate/hyaluronic acid nanoparticles to deliver the antibiotic ceftazidime to the eye. The rheological synergism parameter was calculated by calculating the viscosity of the nanoparticles in contact with mucin in different mass proportions. A minimum viscosity was observed when chitosan nanoparticles were placed in contact with mucin. However, the nanoparticles presented mucoadhesion which resulted in good interaction with the ocular mucosa and prolonged release of the antibiotic, and therefore, the nanoparticles can enhance the life span of the drug in the eyes. The nanoparticles did not show cytotoxicity for two cell lines tested (ARPE-19 and HEK 239T). The nanoparticles were also able to preserve the antibacterial activity, thus making them a promising formulations for the administration of ocular drugs with improved mucoadhesive properties.
Pistone et al. [ 115 ] prepared nanoparticles of chitosan, alginate and pectin as potential candidates for the administration of drugs into the oral cavity. The biocompatibility of the formulations was estimated based on the solubility of the nanoparticles in a salivary environment and its cytotoxicity potential was estimated in an oral cell line. Alginate nanoparticles were the most unwavering in the artificial saliva for at least 2 h, whereas pectin and especially chitosan nanoparticles were unstable. However, the chitosan nanoparticles were the most cyto-competitive, whereas alginate and pectin nanoparticles showed cytotoxicity under all tested conditions (concentration and time). The presence of Zn 2+ (cross-linking agent) may be the cause of the observed cytotoxicity. Each formulation presented advantage and limitations for release into the oral cavity, thus necessitating their further refinement.
In addition, Liu et al. [ 116 ] prepared nanoparticles of carboxymethyl chitosan for the release of intra-nasal carbamazepine (CBZ) to bypass the blood–brain barrier membrane, thus increasing the amount of the medication in the brain and refining the treatment efficacy, thereby reducing the systemic drug exposure. The nanoparticles had a mean diameter of 218.76 ± 2.41 nm, encapsulation efficiency of 80% and drug loading of 35%. Concentrations of CBZ remained higher (P < 0.05) in the brain than the plasma over 240 min.
In another example, Jain and Jain [ 117 ] investigated the discharge profile of 5-fluorouracil (5-FU) from hyaluronic acid-coated chitosan nanoparticles into the gut, via oral administration. Release assays in conditions mimicking the transit from the stomach to the colon indicated the release profile of 5-FU which was protected against discharge in the stomach and small intestine. Also, the high local concentration of drugs would be able to increase the exposure time and thus, enhance the capacity for antitumor efficacy and decrease the systemic toxicity in the treatment of colon cancer.
Another biopolymeric material that has been used as a drug delivery is alginate. This biopolymer presents final carboxyl groups, being classified as anionic mucoadhesive polymer and presents greater mucoadhesive strength when compared with cationic and neutral polymers [ 59 , 118 ]. Patil and Devarajan [ 119 ] developed insulin-containing alginate nanoparticles with nicotinamide as a permeation agent in order to lower the serum glucose levels and raise serum insulin levels in diabetic rats. Nanoparticles administered sublingually (5 IU/kg) in the presence of nicotinamide showed high availability pharmacology (> 100%) and bioavailability (> 80%). The fact that NPs are promising carriers of insulin via the sublingual route have been proved in case of the streptozotocin-induced diabetic mouse model by achieving a pharmacological high potential of 20.2% and bio-availability of 24.1% compared to the subcutaneous injection at 1 IU/kg [ 119 ].
Also, Haque et al. [ 120 ] prepared alginate nanoparticles to release venlafaxine (VLF) via intranasal for treatment of depression. The higher blood/brain ratios of the VLF concentration to the alginate nanoparticles administered intra-nasally when compared to the intranasal VLF and VLF solution intravenously indicated the superiority of the nano-formulation in directly transporting the VLF to the brain. In this way, these nanoparticles are promising for the treatment of depression. In another example, Román et al. [ 121 ] prepared alginate microcapsules containing epidermal growth factor bound on its exterior part to target the non-small cell lung cancer cells. Cisplatin (carcinogen drug) was also loaded in the nanoparticles. The addition of EGF significantly increased specificity of carrier systems and presented kinetics of cell death (H460-lung cancer strain) faster than the free drug.
In addition, Garrait et al. [ 122 ] prepared nanoparticles of chitosan containing Amaranth red (AR) and subsequently microencapsulated these nanoparticles in alginate microparticles and studied the release kinetics of this new system in simulated gastric and intestinal fluids. The microparticles had a mean diameter of 285 μm with a homogeneous distribution; it was observed that there was a release of less than 5% of the AR contained in the systems in the gastric pH conditions, whereas the discharge was fast and comprehensive in the intestinal pH conditions. Thus, the carrier showed promise to protect molecules for intestinal release after oral administration.
Costa et al. [ 123 ] prepared chitosan-coated alginate nanoparticles to enhance the permeation of daptomycin into the ocular epithelium aiming for an antibacterial effect. In vitro permeability was assessed using ocular epithelial cell culture models. The antimicrobial activity of nanoencapsulated daptomycin showed potential over the pathogens engaged in bacterial endophthalmitis. Also, the ocular permeability studies demonstrated that with 4 h of treatment from 9 to 12% in total of daptomycin encapsulated in chitosan/alginate nanoparticles, these were able to cross the HCE and ARPE-19 cells. These results indicated that with this system an increasing in the drug retention in the ocular epithelium has occurred.
Xanthan gum
Xanthan gum (XG) is a high molecular weight heteropolysaccharide produced by Xanthomonas campestris . It is a polyanionic polysaccharide and has good bioadhesive properties. Because it is considered non-toxic and non-irritating, xanthan gum is widely used as a pharmaceutical excipient [ 124 ].
Laffleur and Michalek [ 125 ] have prepared a carrier composed of xanthan gum thiolated with l -cysteine to release tannin in the buccal mucosa to treat sialorrhea. Thiolation of xanthan gum resulted in increased adhesion on the buccal mucosa when compared to native xanthan gum. In addition, xanthan gum thiolate has a higher uptake of saliva whereas tannic acid ad-string and dry the oral mucosa. In this way, this system would be an efficient way of reducing the salivary flow of patients with sialorrhea. Angiogenesis is an important feature in regeneration of soft tissues.
Huang et al. [ 126 ] prepared injectable hydrogels composed of aldehyde-modified xanthan and carboxymethyl-modified chitosan containing potent angiogenic factor (antivascular endothelial growth factor, VEGF) to improve abdominal wall reconstruction. The hydrogel presented release properties mainly in tissues like digestive tract and open wounds. The hydrogel containing VEGF was able to accelerate the angiogenesis process and rebuild the abdominal wall. Menzel et al. [ 127 ] studied a new excipient aiming the use as nasal release system. Xanthan gum was used as a major polymer in which the-((2-amino-2-carboxyethyl) disulfanyl) nicotinic acid (Cys-MNA) was coupled. Characteristics, such as amount of the associated binder, mucoadhesive properties and stability against degradation, were analyzed in the resulting conjugate. Each gram of polymer was ligated with 252.52 ± 20.54 μmol of the binder. The muco-adhesion of the grafted polymer was 1.7 fold greater than that of thiolated xanthan and 2.5 fold greater than, that of native xanthan. In addition, the frequency of ciliary beating of nasal epithelial cells was poorly affected and was reversible only upon the removal of the polymer from the mucosa.
Cellulose and its derivatives are extensively utilized in the drug delivery systems basically for modification of the solubility and gelation of the drugs that resulted in the control of the release profile of the same [ 128 ]. Elseoud et al. [ 129 ] investigated the utilization of cellulose nanocrystals and chitosan nanoparticles for the oral releasing of repaglinide (an anti-hyperglycemic—RPG). The chitosan nanoparticles showed a mean size distribution of 197 nm while the hybrid nanoparticles of chitosan and cellulose nanocrystals containing RPG. Chitosan hybrid nanoparticles and oxidized cellulose nanocrystals containing RPG had a mean diameter of 251–310 nm. The presence of the hydrogen bonds between the cellulose nanocrystals and the drug, resulted in sustained release of the same, and subsequently the nanoparticles made with oxidized cellulose nanocrystals presented lower release when compared to the nanoparticles produced with native cellulose nanocrystals.
Agarwal et al. [ 130 ] have developed a drug targeting mechanism which is based on the conjugation of calcium alginate beads with carboxymethylcellulose (CMC) loaded 5-fluoroacyl (5-FU) and is targeted to the colon. The beads with lower CMC proportions presented greater swelling and muco-adhesiveness in the simulated colonic environment. With existence of colonic enzymes there was a 90% release of 5-FU encapsulated in the beads. Hansen et al. [ 131 ] investigated four cellulose derivatives, including, meteylcellulose, hydroxypropyl methylcellulose, sodium carboxymethylcellulose and cationic hydroxyethyl cellulose for application in drug release into the nasal mucosa. The association of these cellulose derivatives with an additional excipient, was also evaluated. The drug model employed in this process was acyclovir. The viability of the polymers as excipients for nasal release applications was also scrutinized for its ciliary beat frequency (CBF) and its infusion through the tissue system of the nostril cavity. An increase in thermally induced viscosity was observed when the cellulose derivatives were mixed with polymer graft copolymer. Further an increased permeation of acyclovir into the nasal mucosa was detected when it was combined with cationic hydroxyethylcellulose. None of the cellulose derivatives caused negative effects on tissues and cells of the nasal mucosa, as assessed by CBF.
They were discovered by Alec Bangham in 1960. Liposomes are used in the pharmaceutical and cosmetics industry for the transportation of diverse molecules and are among the most studied carrier system for drug delivery. Liposomes are an engrained formulation strategy to improve the drug delivery. They are vesicles of spherical form composed of phospholipids and steroids usually in the 50–450 nm size range [ 132 ]. These are considered as a better drug delivery vehicles since their membrane structure is analogous to the cell membranes and because they facilitate incorporation of drugs in them [ 132 ]. It has also been proved that they make therapeutic compounds stable, improve their biodistribution, can be used with hydrophilic and hydrophobic drugs and are also biocompatible and biodegradable. Liposomes are divided into four types: (1) conventional type liposomes: these consists of a lipid bilayer which can make either anionic, cationic, or neutral cholesterol and phospholipids, which surrounds an aqueous core material. In this case, both the lipid bilayer and the aqueous space can be filled with hydrophobic or hydrophilic materials, respectively. (2) PEGylated types: polyethylene glycol (PEG) is incorporated to the surface of liposome to achieve steric equilibrium, (3) ligand-targeted type: ligands like antibodies, carbohydrates and peptides, are linked to the surface of the liposome or to the end of previously attached PEG chains and (4) theranostic liposome type: it is an amalgamation kind of the previous three types of liposomes and generally consists of a nanoparticle along with a targeting, imaging and a therapeutic element [ 133 ].
The typical synthesis procedure for liposomes are as follows, thin layer hydration, mechanical agitation, solvent evaporation, solvent injection and the surfactant solubilization [ 134 ]. One aspect to point out on liposomes is that the drugs that are trapped within them are not bioavailable until they are released. Therefore, their accumulation in particular sites is very important to increase drug bioavailability within the therapeutic window at the right rates and times. Drug loading in liposomes is attained by active (drug encapsulated after liposome formation) and passive (drug encapsulated during liposome formation) approaches [ 135 ]. Hydrophilic drugs such as ampicillin and, 5-fluoro-deoxyuridine are typically confined in the aqueous core of the liposome and thus, their encapsulation does not depend on any modification in the drug/lipid ratio. However, the hydrophobic ones such as Amphotericin B, Indomethacin were found in the acyl hydrocarbon chain of the liposome and thus their engulfing are subjected to the characteristics of the acyl chain [ 136 ]. Among the passive loading approaches the mechanical and the solvent dispersion method as well as the detergent removal method can be mentioned [ 135 ].
There are obstacles with the use of liposomes for drug delivery purposes in the form of the RES (reticuloendothelial system), opsonization and immunogenicity although there are factors like enhanced permeability and EPR (retention effect) that can be utilized in order to boost the drug delivery efficiency of the liposomes [ 133 , 135 ]. Once liposomes get into the body, they run into opsonins and high density lipoproteins (HDLs) and low density lipoproteins (LDLs) while circulating in the bloodstream by themselves. Opsonins (immunoglobulins and fibronectin, for example) assist RES on recognizing and eliminating liposomes. HDLs and LDLs have interactions with liposomes and decrease their stability. Liposomes tends to gather more in the sites like the liver and the spleen, this is an advantage because then a high concentration of liposomes can help treat pathogenic diseases, although in the case of cancers this can lead to a delay in the removal of lipophilic anticancer drugs. This is the reason why as mentioned at the beginning, different types of liposomes have been developed, in this case PEGylated ones. Dimov et al. [ 137 ] reported an incessant procedure of flow system for the synthesis, functionalization and cleansing of liposomes. This research consists of vesicles under 300 nm in a lab-on-chip that are useful and potential candidates for cost-intensive drugs or protein encapsulation development [ 137 ]. This is very important because costs of production also determine whether or not a specific drug can be commercialized. Liposome-based systems have now been permitted by the FDA [ 133 , 135 , 138 , 139 , 140 ].
Polymeric micelles
Polymeric micelles are nanostructures made of amphiphilic block copolymers that gather by itself to form a core shell structure in the aqueous solution. The hydrophobic core can be loaded with hydrophobic drugs (e.g. camptothecin, docetaxel, paclitaxel), at the same time the hydrophilic shell makes the whole system soluble in water and stabilizes the core. Polymeric micelles are under 100 nm in size and normally have a narrow distribution to avoid fast renal excretion, thus permitting their accumulation in tumor tissues through the EPR effect. In addition, their polymeric shell restrains nonspecific interactions with biological components. These nanostructures have a strong prospective for hydrophobic drug delivery since their interior core structure permits the assimilation of these kind of drugs resulting in enhancement of stability and bioavailability [ 141 , 142 ].
Polymeric micelles are synthesized by two approaches: (1) convenient solvent-based direct dissolution of polymer followed by dialysis process or (2) precipitation of one block by adding a solvent [ 142 , 143 ]. The factors like, hydrophobic chain size in the amphiphilic molecule, amphiphiles concentration, solvent system and temperature, affects the micelle formation [ 144 ]. The micelle assembly creation starts when minimum concentration known as the critical micelle concentration (CMC) is reached by the amphiphilic molecules [ 143 ]. At lower concentrations, the amphiphilic molecules are indeed small and occur independently [ 143 ]. Drugs are loaded within polymeric micelles by three common methodologies such as direct dissolution process, solvent evaporation process, and the dialysis process. As of the direct dissolution process, the copolymer and the drugs combine with each other by themselves in the water medium and forms a drug loaded with the micelles. While in the solvent evaporation process, the copolymer and the intended drug is dissolved using a volatile organic solvent and finally, in case of the dialysis process, both the drug in solution and the copolymer in the organic solvent are combined in the dialysis bag and then dialyzed with the formation of the micelle [ 145 ].
The targeting of the drugs using different polymeric micelles as established by various mechanism of action including the boosted penetrability and the holding effect stimuli; complexing of a definite aiming ligand molecule to the surface of the micelle; or by combination of the monoclonal antibodies to the micelle corona [ 146 ]. Polymeric micelles are reported to be applicable for both drug delivery against cancer [ 143 ] and also for ocular drug delivery [ 147 ] as shown in Fig. 3 in which a polymeric micelle is used for reaching the posterior ocular tissues [ 147 ]. In the work by Li et al. [ 148 ], dasatinib was encapsulated within nanoparticles prepared from micellation of PEG-b-PC, to treat proliferative vitreoretinopathy (PVR), their size was 55 nm with a narrow distribution and they turned out to be noncytotoxic to ARPE-19 cells. This micellar formulation ominously repressed the cell proliferation, attachment and relocation in comparison to the free drugs [ 148 ]. The polymeric micelles is habitually get into the rear eye tissues through the transcleral pathway after relevant applications (Fig. 3 ; [ 147 ]).
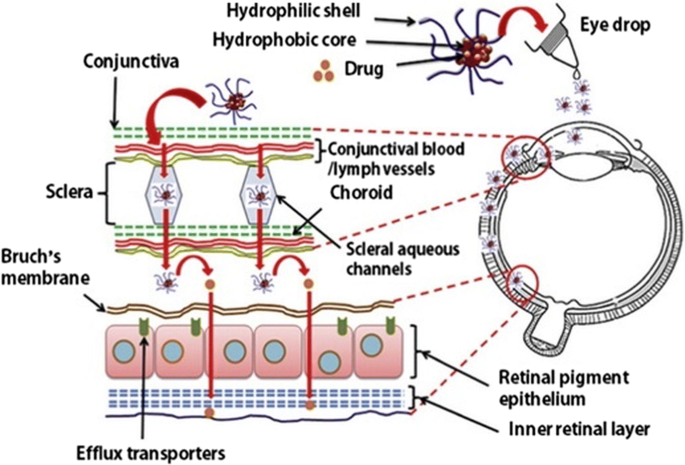
(the figure is reproduced from Mandal et al. [ 147 ] with required copyright permission)
Polymeric micelles used for reaching the posterior ocular tissues via the transcleral pathway after topical application
Dendrimers are highly bifurcated, monodisperse, well-defined and three-dimensional structures. They are globular-shaped and their surface is functionalized easily in a controlled way, which makes these structures excellent candidates as drug delivery agents [ 149 , 150 , 151 ]. Dendrimers can be synthesized by means of two approaches: The first one is the different route in which the dendrimer starts formation from its core and then it is extended outwards and the second is the convergent one, starts from the outside of the dendrimer [ 152 ]. Dendrimers are grouped into several kinds according to their functionalization moieties: PAMAM, PPI, liquid crystalline, core–shell, chiral, peptide, glycodendrimers and PAMAMOS, being PAMAM, the most studied for oral drug delivery because it is water soluble and it can pass through the epithelial tissue boosting their transfer via the paracellular pathway [ 153 ]. Dendrimers are limited in their clinical applications because of the presence of amine groups. These groups are positively charged or cationic which makes them toxic, hence dendrimers are usually modified in order to reduce this toxicity issue or to eliminate it. Drug loading in dendrimers is performed via the following mechanisms: Simple encapsulation, electrostatic interaction and covalent conjugation [ 154 ].
Drug is basically delivered by the dendrimers following two different paths, a) by the in vivo degradation of drug dendrimer’s covalent bonding on the basis of availability of suitable enzymes or favorable environment that could cleave the bonds and b) by discharge of the drug due to changes in the physical environment like pH, temperature etc., [ 154 ]. Dendrimers have been developed for transdermal, oral, ocular, pulmonary and in targeted drug delivery [ 155 ].
Jain et al. [ 156 ] have described the folate attached poly- l -lysine dendrimers (doxorubicin hydrochloride) as a capable cancer prevention drug carrier model for pH dependent drug discharge, target specificity, antiangiogenic and anticancer prospective, it was shown that doxorubicin-folate conjugated poly- l -lysine dendrimers increased the concentration of doxorubicin in the tumor by 121.5-fold after 24 h compared with free doxorubicin. Similarly, (Kaur et al. [ 157 ] developed folate-conjugated polypropylene imine dendrimers (FA-PPI) as a methotrexate (MTX) nanocarrier, for pH-sensitive drug release, selective targeting to cancer cells, and anticancer treatment. The in vitro studies on them showed sustained release, increased cell uptake and low cytotoxicity on MCF-7 cell lines [ 157 ]. Further, it has to be pointed out that the developed formulations, methotrexate (MTX)-loaded and folic acid-conjugated 5.0G PPI (MTX-FA-PPI), were selectively taken up by the tumor cells in comparison with the free drug, methotrexate (MTX).
Inorganic nanoparticles
Inorganic nanoparticles include silver, gold, iron oxide and silica nanoparticles are included. Studies focused on them are not as many as there are on other nanoparticle types discussed in this section although they show some potential applications. However, only few of the nanoparticles have been accepted for its clinical use, whereas the majority of them are still in the clinical trial stage. Metal nanoparticles, silver and gold, have particular properties like SPR (surface plasmon resonance), that liposomes, dendrimers, micelles do not possess. They showed several advantages such as good biocompatibility and versatility when it comes to surface functionalization.
Studies on their drug delivery-related activity have not been able to clear out whether the particulate or ionized form is actually related to their toxicity, and even though two mechanisms have been proposed, namely paracellular transport and transcytosis, there is not enough information about their in vivo transport and uptake mechanism [ 158 ]. Drugs can be conjugated to gold nanoparticles (AuNPs) surfaces via ionic or covalent bonding and physical absorption and they can deliver them and control their release through biological stimuli or light activation [ 159 ]. Silver nanoparticles exhibited antimicrobial activity, but as for drug delivery, very few studies have been carried out, for example, Prusty and Swain [ 160 ] synthesized an inter-linked and spongy polyacrylamide/dextran nano-hydrogels hybrid system with covalently attached silver nanoparticles for the release of ornidazole which turned out to have an in vitro release of 98.5% [ 160 ]. Similarly in another study, the iron oxide nanoparticles were synthesized using laser pyrolysis method and were covered with Violamycine B1, and antracyclinic antibiotics and tested against the MCF-7 cells for its cytotoxicity and the anti-proliferation properties along with its comparison with the commercially available iron oxide nanoparticles [ 161 ].
Nanocrystals
Nanocrystals are pure solid drug particles within 1000 nm range. These are 100% drug without any carriers molecule attached to it and are usually stabilized by using a polymeric steric stabilizers or surfactants. A nanocrystals suspension in a marginal liquid medium is normally alleviated by addition of a surfactant agent known as nano-suspension. In this case, the dispersing medium are mostly water or any aqueous or non-aqueous media including liquid polyethylene glycol and oils [ 162 , 163 ]. Nanocrystals possesses specific characters that permit them to overcome difficulties like increase saturation solubility, increased dissolution velocity and increased glueyness to surface/cell membranes. The process by which nanocrystals are synthesized are divided into top-down and bottom-up approaches. The top-down approach includes, sono-crystallization, precipitation, high gravity controlled precipitation technology, multi-inlet vortex mixing techniques and limited impinging liquid jet precipitation technique [ 162 ]. However, use of an organic solvent and its removal at the end makes this process quite expensive. The bottom-up approach involves, grinding procedures along with homogenization at higher pressure [ 162 ]. Among all of the methods, milling, high pressure homogenization, and precipitation are the most used methods for the production of nanocrystals. The mechanisms by which nanocrystals support the absorption of a drug to the system includes, enhancement of solubility, suspension rate and capacity to hold intestinal wall firmly [ 162 ]. Ni et al. [ 164 ] embedded cinaciguat nanocrystals in chitosan microparticles for pulmonary drug delivery of the hydrophobic drug. The nanoparticles were contrived for continuous release of the drug taking advantage of the swelling and muco-adhesive potential of the polymer. They found that inhalation efficacy might be conceded under the disease conditions, so more studies are needed to prove that this system has more potential [ 164 ].
Metallic nanoparticles
In recent years, the interest of using metallic nanoparticles has been growing in different medical applications, such as bioimaging, biosensors, target/sustained drug delivery, hyperthermia and photoablation therapy [ 35 , 165 ]. In addition, the modification and functionalization of these nanoparticles with specific functional groups allow them to bind to antibodies, drugs and other ligands, become these making these systems more promising in biomedical applications [ 166 ]. Although the most extensively studied, metallic nanoparticles are gold, silver, iron and copper, a crescent interest has been exploited regarding other kinds of metallic nanoparticles, such as, zinc oxide, titanium oxide, platinum, selenium, gadolinium, palladium, cerium dioxide among others [ 35 , 165 , 166 ].
Quantum dots
Quantum dots (QDs) are known as semiconductor nanocrystals with diameter range from 2 to 10 nm and their optical properties, such as absorbance and photoluminescence are size-dependent [ 167 ]. The QDs has gained great attention in the field of nanomedicine, since, unlike conventional organic dyes, the QDs presents emission in the near-infrared region (< 650 nm), a very desirable characteristic in the field of biomedical images, due to the low absorption by the tissues and reduction in the light scattering [ 167 , 168 ]. In addition, QDs with different sizes and/or compositions can be excited by the same light source resulting in separate emission colors over a wide spectral range [ 169 , 170 ]. In this sense, QDs are very appealing for multiplex imaging. In the medicine field QDs has been extensively studied as targeted drug delivery, sensors and bioimaging. A large number of studies regarding the applications of QDs as contrast agents for in vivo imaging is currently available in literature [ 168 , 171 , 172 , 173 ]. Han et al. [ 172 ] developed a novel fluorophore for intravital cytometric imaging based on QDs-antibodies conjugates coated with norbornene-displaying polyimidazole ligands. This fluorophore was used to label bone marrow cells in vivo. The authors found that the fluorophore was able to diffuse in the entire bone marrow and label rare populations of cells, such as hematopoietic stem and progenitor cells [ 172 ]. Shi et al. [ 171 ] developed a multifunctional biocompatible graphene oxide quantum dot covered with luminescent magnetic nanoplatform for recognize/diagnostic of a specific liver cancer tumor cells (glypican-3-expressing Hep G2). According to the authors the attachment of an anti-GPC3-antibody to the nanoplataform results in selective separation of Hep G2 hepatocellular carcinoma cells from infected blood samples [ 171 ]. QDs could also bring benefits in the sustained and/or controlled release of therapeutic molecules. Regarding the controlled release, this behavior can be achieved via external stimulation by light, heat, radio frequency or magnetic fields [ 170 , 174 , 175 ]. Olerile et al. [ 176 ] have developed a theranostic system based on co-loaded of QDs and anti-cancer drug in nanostructured lipid carriers as a parenteral multifunctional system. The nanoparticles were spherical with higher encapsulation efficiency of paclitaxel (80.7 ± 2.11%) and tumor growth inhibition rate of 77.85%. The authors also found that the system was able to specifically target and detect H22 tumor cells [ 176 ]. Cai et al. [ 177 ] have synthesized pH responsive quantum dots based on ZnO quantum dots decorated with PEG and hyaluronic acid for become stable in physiological conditions and for targeting specific cells with HA-receptor CD44, respectively. This nanocarrier was also evaluated for doxorubicin (DOX) sustained release. The nanocarrier was stable in physiological pH and DOX was loaded in the carrier by forming complex with Zn 2+ ions or conjugated to PEG. The DOX was released only in acidic intracellular conditions of tumor cells due to the disruption of ZnO QDs. The authors found that the anticancer activity was enhanced by the combination of DOX and ZnO QDs [ 177 ].
Protein and polysaccharides nanoparticles
Polysaccharides and proteins are collectively called as natural biopolymers and are extracted from biological sources such as plants, animals, microorganisms and marine sources [ 178 , 179 ]. Protein-based nanoparticles are generally decomposable, metabolizable, and are easy to functionalize for its attachment to specific drugs and other targeting ligands. They are normally produced by using two different systems, (a) from water-soluble proteins like bovine and human serum albumin and (b) from insoluble ones like zein and gliadin [ 180 ]. The usual methods to synthesize them are coacervation/desolvation, emulsion/solvent extraction, complex coacervation and electrospraying. The protein based nanoparticles are chemically altered in order to combine targeting ligands that identify exact cells and tissues to promote and augment their targeting mechanism [ 180 ]. Similarly, the polysaccharides are composed of sugar units (monosaccharides) linked through O-glycosidic bonds. The composition of these monomers as well as their biological source are able to confer to these polysaccharides, a series of specific physical–chemical properties [ 126 , 179 , 181 ]. One of the main drawback of the use of polysaccharides in the nanomedicine field is its degradation (oxidation) characteristics at high temperatures (above their melting point) which are often required in industrial processes. Besides, most of the polysaccharides are soluble in water, which limits their application in some fields of nanomedicine, such as tissue engineering [ 182 , 183 ]. However, techniques such as crosslinking of the polymer chains have been employed in order to guarantee stability of the polysaccharide chains, guaranteeing them stability in aqueous environments [ 182 , 183 ]. In Fig. 4 , examples of some polysaccharides used in nanomedicine obtained from different sources are summarized. The success of these biopolymers in nanomedicine and drug delivery is due to their versatility and specified properties such as since they can originate from soft gels, flexible fibers and hard shapes, so they can be porous or non-porous; they have great similarity with components of the extracellular matrix, which may be able to avoid immunological reactions [ 179 , 184 ].
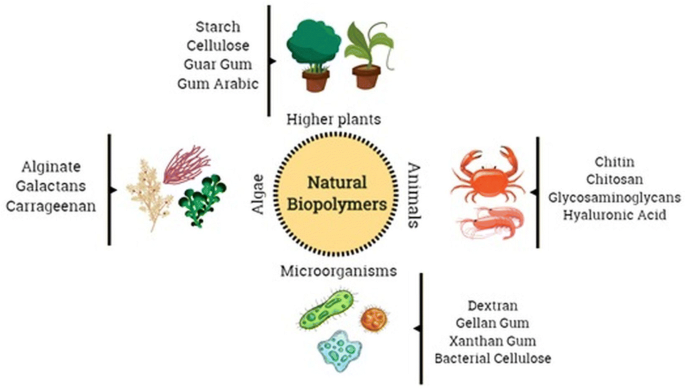
Different sources of natural biopolymers to be used in nanomedicine applications. Natural biopolymers could be obtained from higher plants, animals, microorganisms and algae
There is not much literature related to these kind of nanoparticles, however, since they are generated from biocompatible compounds they are excellent candidates for their further development as drug delivery systems. Yu et al. [ 185 ] synthesized Bovine serum albumin and tested its attachment and/or infiltration property through the opening of the cochlea and middle ear of guinea pigs. The nanoparticles considered as the drug transporters were tested for their loading capacity and release behaviors that could provide better bio-suitability, drug loading capacity, and well-ordered discharge mechanism [ 185 ].
Natural product-based nanotechnology and drug delivery
As per the World Health Organization (WHO) report, in developing countries, the basic health needs of approximately 80% of the population are met and/or complemented by traditional medicine [ 186 ]. Currently, the scientific community is focusing on the studies related to the bioactive compounds, its chemical composition and pharmacological potential of various plant species, to produce innovative active ingredients that present relatively minor side effects than existing molecules [ 5 , 187 ]. Plants are documented as a huge sources of natural compounds of medicinal importance since long time and still it holds ample of resources for the discovery of new and highly effective drugs. However, the discovery of active compounds through natural sources is associated with several issues because they originate from living beings whose metabolite composition changes in the presence of stress. In this sense, the pharmaceutical industries have chosen to combine their efforts in the development of synthetic compounds [ 187 , 188 , 189 ]. Nevertheless, the number of synthetic molecules that are actually marketed are going on decreasing day by day and thus research on the natural product based active compounds are again coming to the limelight in spite of its hurdles [ 189 , 190 ]. Most of the natural compounds of economic importance with medicinal potential that are already being marketed have been discovered in higher plants [ 187 , 191 ]. Several drugs that also possess natural therapeutic agents in their composition are already available commercially; their applications and names are as follows: malaria treatment (Artemotil ® derived from Artemisia annua L., a traditional Chinese medicine plant), Alzheimer’s disease treatment (Reminyl ® , an acetylcholinesterase inhibitor isolated from the Galanthus woronowii Losinsk), cancer treatment (Paclitaxel ® and its analogues derived from the Taxus brevifolia plant; vinblastine and vincristine extracted from Catharanthus roseus ; camptothecin and its analogs derived from Camptotheca acuminata Decne), liver disease treatment (silymarin from Silybum marianum ) [ 187 ].
The composition and activity of many natural compounds have already been studied and established. The alkaloids, flavonoids, tannins, terpenes, saponins, steroids, phenolic compounds, among others, are the bioactive molecules found in plants. However in most of the cases, these compounds have low absorption capacity due to the absence of the ability to cross the lipid membranes because of its high molecular sizes, and thus resulting in reduced bioavailability and efficacy [ 192 ]. These molecules also exhibit high systemic clearance, necessitating repeated applications and/or high doses, making the drug less effective for therapeutic use [ 189 ]. The scientific development of nanotechnology can revolutionize the development of formulations based on natural products, bringing tools capable of solving the problems mentioned above that limits the application of these compounds in large scale in the nanomedicine [ 7 , 189 ]. Utilization of nanotechnology techniques in the medical field has been extensively studied in the last few years [ 193 , 194 ]. Hence these can overcome these barriers and allow different compounds and mixtures to be used in the preparation of the same formulation. In addition, they can change the properties and behavior of a compound within the biological system [ 7 , 189 ]. Besides, bringing benefits to the compound relative to the solubility and stability of the compounds, release systems direct the compound to the specific site, increase bioavailability and extend compound action, and combine molecules with varying degrees of hydrophilicity/lipophilicity [ 7 ]. Also, there is evidence that the association of release systems with natural compounds may help to delay the development of drug resistance and therefore plays an important role in order to find new possibilities for the treatment of several diseases that have low response to treatment conventional approaches to modern medicine [ 7 , 189 ].
The natural product based materials are of two categories, (1) which are targeted to specific location and released in the specific sites to treat a number of diseases [ 43 , 195 ] and (2) which are mostly utilized in the synthesis process [ 196 ]. Most of the research is intended for treatment against the cancer disease, since it is the foremost reason of death worldwide nowadays [ 197 , 198 ]. In case of the cancer disease, different organs of the body are affected, and therefore the need for the development of an alternative medicine to target the cancerous cells is the utmost priority among the modern researchers, however, a number of applications of nanomedicine to other ailments is also being worked on [ 199 , 200 ]. These delivery systems are categorized in terms of their surface charge, particle size, size dispersion, shape, stability, encapsulation potential and biological action which are further utilized as per their requirements [ 33 ]. Some examples of biological compounds obtained from higher plants and their uses in the nanomedicine field are described in Fig. 5 . Pharmaceutical industries have continuously sought the development and application of new technologies for the advancement and design of modern drugs, as well as the enhancement of existing ones [ 71 , 201 ]. In this sense, the accelerated development of nanotechnology has driven the design of new formulations through different approaches, such as, driving the drug to the site of action (nanopharmaceutics); image and diagnosis (nanodiagnostic), medical implants (nanobiomaterials) and the combination diagnosis and treatment of diseases (nanotheranostics) [ 71 , 202 , 203 ].
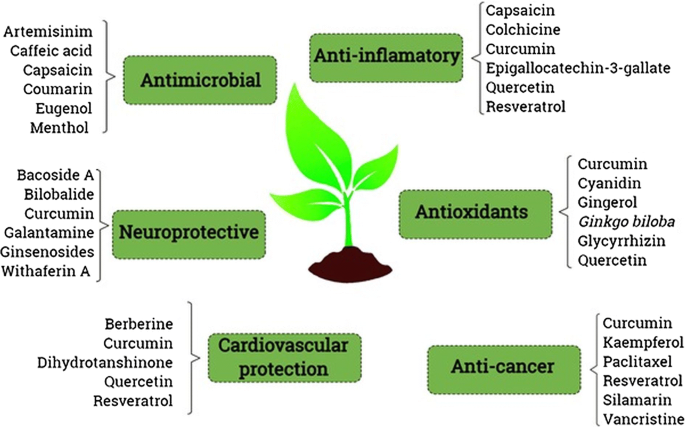
Examples of natural compounds extracted from higher plants used in nanomedicine aiming different approaches. Some of these extracts are already being marketed, others are in clinical trials and others are being extensively studied by the scientific community
Currently, many of the nanomedicines under development, are modified release systems for active ingredients (AI) that are already employed in the treatment of patients [ 203 , 204 ]. For this type of approach, it is evaluated whether the sustained release of these AIs modifies the pharmacokinetic profile and biodistribution. In this context, it can be ascertained that the nano-formulation offers advantages over the existing formulation if the AI is directed towards the target tissue shows increased uptake/absorption by the cells and lower toxicity profile for the organism [ 205 , 206 ]. This section is focused on berberine, curcumin, ellagic acid, resveratrol, curcumin and quercetin [ 8 ]. Some other compounds mentioned are doxorubicin, paclitaxel and vancomycin that also come from natural products.
Nanoparticles have been synthesized using natural products. For example, metallic, metal oxide and sulfides nanoparticles have been reported to be synthesized using various microorganisms including bacteria, fungi, algae, yeast and so on [ 207 ] or plant extracts [ 208 ]. For the first approach, the microorganism that aids the synthesis procedure is prepared in the adequate growth medium and then mixed with a metal precursor in solution and left for incubation to form the nanoparticles either intracellularly or extracellularly [ 209 , 210 , 211 ]. As for the second approach, the plant extract is prepared and mixed afterwards with the metal precursor in solution and incubated further at room temperature or boiling temperature for a definite time or exposed to light as an external stimulus to initiate the synthesis of nanoparticles [ 212 ].
Presently, these natural product based materials are considered as the key ingredients in the preparation and processing of new nano-formulations because they have interesting characteristics, such as being biodegradable, biocompatible, availability, being renewable and presenting low toxicity [ 178 , 179 , 213 ]. In addition to the aforementioned properties, biomaterials are, for the most part, capable of undergoing chemical modifications, guaranteeing them unique and desirable properties for is potential uses in the field of nanomedicine [ 45 , 214 ]. Gold, silver, cadmium sulfide and titanium dioxide of different morphological characteristics have been synthesized using a number of bacteria namely Escherichia coli , Pseudomonas aeruginosa , Bacillus subtilis and Klebsiella pneumoniae [ 211 ]. These nanoparticles, especially the silver nanoparticles have been abundantly studied in vitro for their antibacterial, antifungal, and cytotoxicity potential due to their higher potential among all metal nanoparticles [ 215 , 216 ]. In the event of microorganism mediated nanoparticle synthesis, maximum research is focused on the way that microorganisms reduce metal precursors and generate the nanoparticles. For instance, Rahimi et al. [ 217 ] synthesized silver nanoparticles using Candida albicans and studied their antibacterial activity against two pathogenic bacteria namely Staphylococcus aureus and E. coli. Similarly, Ali et al. [ 218 ] synthesized silver nanoparticles with the Artemisia absinthium aqueous extract and their antimicrobial activity was assessed versus Phytophthora parasitica and Phytophthora capsici [ 218 ]. Further, Malapermal et al. [ 219 ] used Ocimum basilicum and Ocimum sanctum extracts to synthesize nanoparticles and studied its antimicrobial potential against E. coli , Salmonella spp., S. aureus , and P. aeruginosa along with the antidiabetic potential. Likewise, Sankar et al. [ 220 ] also tested the effect of silver nanoparticles for both antibacterial and anticancer potential against human lung cancer cell line. Besides the use of microorganism, our group has synthesized silver, gold and iron oxide nanoparticles using various food waste materials such as extracts of Zea mays leaves [ 221 , 222 ], onion peel extract [ 223 ], silky hairs of Zea mays [ 224 ], outer peel of fruit of Cucumis melo and Prunus persica [ 225 ], outer peel of Prunus persica [ 226 ] and the rind extract of watermelon [ 227 ], etc. and have tested their potential antibacterial effects against various foodborne pathogenic bacteria, anticandidal activity against a number of pathogenic Candida spp., for their potential antioxidant activity and proteasome inhibitory effects.
For drug delivery purposes, the most commonly studied nanocarriers are crystal nanoparticles, liposomes, micelles, polymeric nanoparticles, solid lipid nanoparticles, superparamagnetic iron oxide nanoparticles and dendrimers [ 228 , 229 , 230 ]. All of these nanocarriers are formulated for natural product based drug delivery. For applications in cancer treatment, Gupta et al. [ 231 ] synthesized chitosan based nanoparticles loaded with Paclitaxel (Taxol) derived from Taxus brevifolia , and utilized them for treatment of different kinds of cancer. The authors concluded that the nanoparticle loaded drug exhibited better activity with sustained release, high cell uptake and reduced hemolytic toxicity compared with pure Paclitaxel [ 231 ]. Berberine is an alkaloid from the barberry plant. Chang et al. [ 232 ] created a heparin/berberine conjugate to increase the suppressive Helicobacter pylori growth and at the same time to reduce cytotoxic effects in infected cells [ 232 ] which is depicted in Fig. 6 .
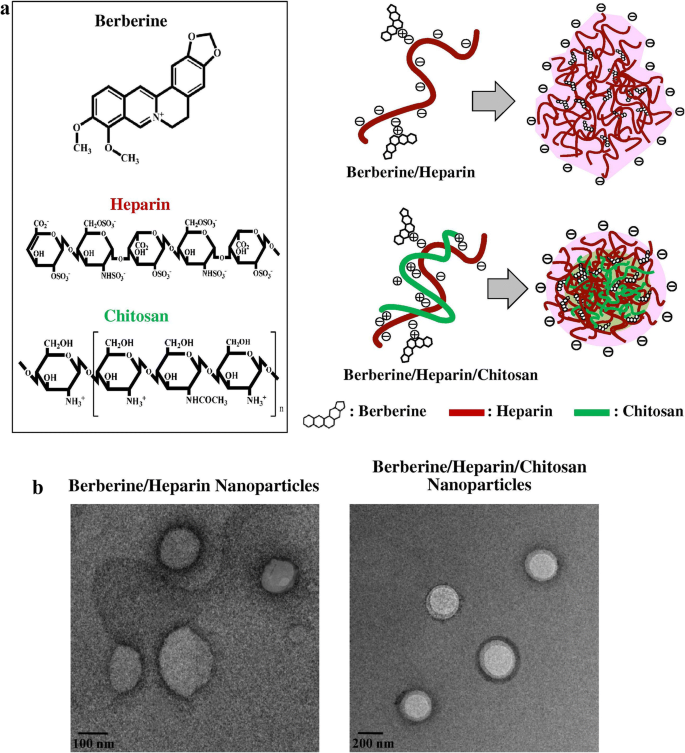
(the figure is reproduced from Chang et al. [ 232 ] with required copyright permission)
a Structure of berberine/heparin based nanoparticles and berberine/heparin/chitosan nanoparticles. b TEM images of the berberine/heparin nanoparticles and berberine/heparin/chitosan nanoparticles
Aldawsari and Hosny [ 233 ] synthesized ellagic acid-SLNs to encapsulate Vancomycin (a glycopeptide antibiotic produced in the cultures of Amycolatopsis orientalis ). Further, its in vivo tests were performed on rabbits and the results indicated that the ellagic acid prevented the formation of free oxygen radicals and their clearance radicals, thus preventing damages and promoting repair [ 233 ]. Quercetin is a polyphenol that belongs to the flavonoid group, it can be found in citrus fruits and vegetables and it has antioxidant properties. In a study by Dian et al. [ 234 ], polymeric micelles was used to deliver quercetin and the results showed that such micelles could provide continuous release for up to 10 days in vitro, with continuous plasma level and boosted complete accessibility of the drug under in vivo condition [ 234 ].
Daunorubicin is a natural product derived from a number of different wild type strains of Streptomyces , doxorubicin (DOX) is a hydrolated version of it used in chemotherapy [ 213 ]. Spillmann et al. [ 235 ] developed a multifunctional liquid crystal nanoparticle system for intracellular fluorescent imaging and for the delivery of doxorubicin in which the nanoparticles were functionalized with transferrin. Cellular uptake and sustained released were attained within endocytic vesicles in HEK 293T/17 cells. Perylene was used as a chromophore to track the particles and to encapsulate agents aimed for intracellular delivery [ 235 ]. Purama et al. [ 236 ] extracted dextran from two sucrose based lactic acid bacteria namely Streptococcus mutans and Leuconostoc mesenteroides . Agarwal et al. [ 237 ] formulated a dextran-based dendrimer formulation and evaluated its drug discharge capacity and haemolytic activity under in vitro condition. They concluded that the dendritic structure selectively enters the highly permeable portion of the affected cells without disturbing the healthy tissues thereby making more convenient for its application in the biomedical field [ 237 ]. Folate- functionalized superparamagnetic iron oxide nanoparticles developed previously for liver cancer cure are also been used for the delivery of Doxil (a form of doxorubicin which was the first FDA-approved nano-drug in 1995) [ 238 ]. The in vivo studies in rabbits and rats showed a two- and fourfold decrease compared with Doxil alone while folate aided and enhanced specific targeting [ 239 ]. Liposomes are the nanostructures that have been studied the most, and they have been used in several formulations for the delivery of natural products like resveratrol [ 240 ]. Curcumin, a polyphenolic compound obtained from turmeric, have been reported to be utilized in the cure of cancers including the breast, bone, cervices, liver, lung, and prostate [ 241 ]. Liposomal curcumin formulations have been developed for the treatment of cancer [ 242 , 243 ]. Cheng et al. [ 244 ] encapsulated curcumin in liposomes by different methods and compared the outcomes resulting that the one dependent on pH yielded stable products with good encapsulation efficiency and bio-accessibility with potential applications in cancer treatment [ 244 ].
Over all, it can be said that the sustained release systems of naturally occurring therapeutic compounds present themselves as a key tools for improving the biological activity of these compounds as well as minimizing their limitations by providing new alternatives for the cure of chronic and terminal diseases [ 8 , 245 ]. According to BBC Research, the global market for plant-derived pharmaceuticals will increase from $29.4 billion in 2017 to about $39.6 billion in 2022 with a compound annual growth rate (CAGR) of 6.15% in this period (BCC-RESEARCH). Some of nanostructure-based materials covered in this section have already been approved by the FDA. Bobo et al. [ 255 ] has provided the information on nanotechnology-based products already approved by the FDA (Table 1 ).
Regulation and reality: products now on the market
In the current medical nanotechnology scenario, there are 51 products based on this technology [ 204 , 246 , 247 , 248 ] which are currently being applied in clinical practice (Table 2 ). Notably, such nanomedicines are primarily developed for drugs, which have low aqueous solubility and high toxicity, and these nanoformulations are often capable of reducing the toxicity while increasing the pharmacokinetic properties of the drug in question.
According to a recent review by Caster et al. [ 249 ], although few nanomedicines have been regulated by the FDA there are many initiatives that are currently in progress in terms of clinical trials suggesting many nanotechnology-based new drugs will soon be able to reach the market. Among these nanomaterials that are in phase of study, 18 are directed to chemotherapeutics; 15 are intended for antimicrobial agents; 28 are for different medical applications and psychological diseases, autoimmune conditions and many others and 30 are aimed at nucleic acid based therapies [ 249 ]. The list of nanomedicine approved by FDA classified by type of carrier/material used in preparation of the formulation is shown in Table 2 .
Nanotechnology has dynamically developed in recent years, and all countries, whether developed or not, are increasing their investments in research and development in this field. However, researchers who work with practical applications of the nano-drugs deal with high levels of uncertainties, such as a framing a clear definition of these products; characterization of these nanomaterials in relation to safety and toxicity; and the lack of effective regulation. Although the list of approved nanomedicine is quite extensive, the insufficiency of specific regulatory guidelines for the development and characterization of these nanomaterials end up hampering its clinical potential [ 250 ]. The structure/function relationships of various nanomaterials, as well as their characteristics, composition and surface coating, interacts with the biological systems. In addition, it is important to evaluate the possibility of aggregate and agglomerate formation when these nanomedicines are introduced into biological systems, since they do not reflect the properties of the individual particle; this may generate different results and/or unexpected toxic effects depending on the nano-formulation [ 250 ].
The lack of standard protocols for nanomedicines characterization at physico-chemical and physiological/biological levels has often limited the efforts of many researchers to determine the toxic potential of nano-drugs in the early stages of testing, and that resulted in the failures in late-phase clinical trials. To simplify and/or shorten the approval process for nano based medicines/drugs, drug delivery system etc., a closer cooperation among regulatory agencies is warranted [ 204 , 251 ].
As a strategy for the lack of regulation of nanomedicines and nano drug delivery system; the safety assessment and the toxicity and compatibility of these are performed based on the regulations used by the FDA for conventional drugs. After gaining the status of a new research drug (Investigational New Drug, IND) by the FDA, nanomedicines, nano-drug delivery systems begin the clinical trials phase to investigate their safety and efficacy in humans. These clinical trials are divided into three phases: phase 1 (mainly assesses safety); phase 2 (mainly evaluates efficacy) and phase 3 (safety, efficacy and dosage are evaluated). After approval in these three phases the IND can be filed by the FDA to request endorsement of the new nanomedicine or nano drug delivery systems. However, this approach to nanomedicine regulation has been extensively questioned [ 204 , 246 , 252 ].
Due to the rapid development of nanotechnology as well as its potential use of nanomedicine, a reformed and more integrated regulatory approach is urgently required. In this regard, country governments must come together to develop new protocols that must be specific and sufficiently rigorous to address any safety concerns, thus ensuring the release of safe and beneficial nanomedicine for patients [ 204 , 252 , 253 ].
Future of nanomedicine and drug delivery system
The science of nanomedicine is currently among the most fascinating areas of research. A lot of research in this field in the last two decades has already led to the filling of 1500 patents and completion of several dozens of clinical trials [ 254 ]. As outlined in the various sections above, cancer appears to be the best example of diseases where both its diagnosis and therapy have benefited from nonmedical technologies. By using various types of nanoparticles for the delivery of the accurate amount of drug to the affected cells such as the cancer/tumour cells, without disturbing the physiology of the normal cells, the application of nanomedicine and nano-drug delivery system is certainly the trend that will remain to be the future arena of research and development for decades to come.
The examples of nanoparticles showed in this communications are not uniform in their size, with some truly measuring in nanometers while others are measured in sub-micrometers (over 100 nm). More research on materials with more consistent uniformity and drug loading and release capacity would be the further area of research. Considerable amount of progress in the use of metals-based nanoparticles for diagnostic purposes has also been addressed in this review. The application of these metals including gold and silver both in diagnosis and therapy is an area of research that could potentially lead to wider application of nanomedicines in the future. One major enthusiasm in this direction includes the gold-nanoparticles that appear to be well absorbed in soft tumour tissues and making the tumour susceptible to radiation (e.g., in the near infrared region) based heat therapy for selective elimination.
Despite the overwhelming understanding of the future prospect of nanomedicine and nano-drug delivery system, its real impact in healthcare system, even in cancer therapy/diagnosis, remains to be very limited. This attributes to the field being a new area of science with only two decades of real research on the subject and many key fundamental attributes still being unknown. The fundamental markers of diseased tissues including key biological markers that allow absolute targeting without altering the normal cellular process is one main future area of research. Ultimately, the application of nanomedicine will advance with our increasing knowledge of diseases at molecular level or that mirrors a nanomaterial-subcellular size comparable marker identification to open up avenues for new diagnosis/therapy. Hence, understanding the molecular signatures of disease in the future will lead to advances in nanomedicine applications. Beyond what we have outlined in this review using the known nanoprobes and nanotheragnostics products, further research would be key for the wider application of nanomedicine.
The concept of controlled release of specific drugs at the beleaguered sites, technology for the assessment of these events, drug’s effect in tissues/cellular level, as well as theoretical mathematical models of predication have not yet been perfected. Numerous studies in nanomedicine areas are centered in biomaterials and formulation studies that appear to be the initial stages of the biomedicine applications. Valuable data in potential application as drug therapeutic and diagnosis studies will come from animal studies and multidisciplinary researches that requires significant amount of time and research resources. With the growing global trend to look for more precise medicines and diagnosis, the future for a more intelligent and multi-centered approach of nanomedicine and nano-drug delivery technology looks bright.
There has been lots of enthusiasm with the simplistic view of development of nanorobots (and nanodevices) that function in tissue diagnosis and repair mechanism with full external control mechanism. This has not yet been a reality and remains a futuristic research that perhaps could be attained by mankind in the very near future. As with their benefits, however, the potential risk of nanomedicines both to humans and the environment at large require long term study too. Hence, proper impact analysis of the possible acute or chronic toxicity effects of new nanomaterials on humans and environment must be analyzed. As nanomedicines gain popularity, their affordability would be another area of research that needs more research input. Finally, the regulation of nanomedicines, as elaborated in the previous section will continue to evolve alongside the advances in nanomedicine applications.
The present review discusses the recent advances in nanomedicines, including technological progresses in the delivery of old and new drugs as well as novel diagnostic methodologies. A range of nano-dimensional materials, including nanorobots and nanosensors that are applicable to diagnose, precisely deliver to targets, sense or activate materials in live system have been outlined. Initially, the use of nanotechnology was largely based on enhancing the solubility, absorption, bioavailability, and controlled-release of drugs. Even though the discovery of nanodrugs deal with high levels of uncertainties, and the discovery of pharmacologically active compounds from natural sources is not a favored option today, as compared to some 50 years ago; hence enhancing the efficacy of known natural bioactive compounds through nanotechnology has become a common feature. Good examples are the therapeutic application of nanotechnology for berberine, curcumin, ellagic acid, resveratrol, curcumin and quercetin. The efficacy of these natural products has greatly improved through the use of nanocarriers formulated with gold, silver, cadmium sulphide, and titanium dioxide polymeric nanoparticles together with solid lipid nanoparticles, crystal nanoparticles, liposomes, micelles, superparamagnetic iron oxide nanoparticles and dendrimers.
There has been a continued demand for novel natural biomaterials for their quality of being biodegradable, biocompatible, readily availability, renewable and low toxicity. Beyond identifying such polysaccharides and proteins natural biopolymers, research on making them more stable under industrial processing environment and biological matrix through techniques such as crosslinking is among the most advanced research area nowadays. Polymeric nanoparticles (nanocapsules and nanospheres) synthesized through solvent evaporation, emulsion polymerization and surfactant-free emulsion polymerization have also been widely introduced. One of the great interest in the development of nanomedicine in recent years relates to the integration of therapy and diagnosis (theranostic) as exemplified by cancer as a disease model. Good examples have been encapsulated such as, oleic acid-coated iron oxide nanoparticles for diagnostic applications through near-infrared; photodynamic detection of colorectal cancer using alginate and folic acid based chitosan nanoparticles; utilization of cathepsin B as metastatic processes fluorogenic peptide probes conjugated to glycol chitosan nanoparticles; iron oxide coated hyaluronic acid as a biopolymeric material in cancer therapy; and dextran among others.
Since the 1990s, the list of FDA-approved nanotechnology-based products and clinical trials has staggeringly increased and include synthetic polymer particles; liposome formulations; micellar nanoparticles; protein nanoparticles; nanocrystals and many others often in combination with drugs or biologics. Even though regulatory mechanisms for nanomedicines along with safety/toxicity assessments will be the subject of further development in the future, nanomedicine has already revolutionized the way we discover and administer drugs in biological systems. Thanks to advances in nanomedicine, our ability to diagnose diseases and even combining diagnosis with therapy has also became a reality.
Abbreviations
Amaranth red
ciliary beat frequency
carbamazepine
colorectal cancer
carboxymethylcellulose
((2-amino-2-carboxyethyl) disulfanyl) nicotinic acid (Cys-MNA)
penetrability and holding
folic acid-conjugated dextran
Food and Drug Administration
ferrous oxide
hyaluronic acid
high density lipoproteins
hydroxypropylmethylcellulose
low density lipoproteins
magnetic resonance
near infrared
nanoparticle
perfluorohexane
repaglidine
antivascular endothelial growth factor
venlafaxine
xanthan gum
Swamy MK, Sinniah UR. Patchouli (Pogostemon cablin Benth.): botany, agrotechnology and biotechnological aspects. Ind Crops Prod. 2016;87:161–76.
Article CAS Google Scholar
Mohanty SK, Swamy MK, Sinniah UR, Anuradha M. Leptadenia reticulata (Retz.) Wight & Arn. (Jivanti): botanical, agronomical, phytochemical, pharmacological, and biotechnological aspects. Molecules. 1019;2017:22.
Google Scholar
Rodrigues T, Reker D, Schneider P, Schneider G. Counting on natural products for drug design. Nat Chem. 2016;8:531.
Article CAS PubMed Google Scholar
Siddiqui AA, Iram F, Siddiqui S, Sahu K. Role of natural products in drug discovery process. Int J Drug Dev Res. 2014;6(2):172–204.
CAS Google Scholar
Beutler JA. Natural products as a foundation for drug discovery. Curr Prot Pharmacol. 2009;46(1):9–11.
Thilakarathna SH, Rupasinghe H. Flavonoid bioavailability and attempts for bioavailability enhancement. Nutrients. 2013;5:3367–87.
Article PubMed PubMed Central CAS Google Scholar
Bonifácio BV, da Silva PB, dos Santos Ramos MA, Negri KMS, Bauab TM, Chorilli M. Nanotechnology-based drug delivery systems and herbal medicines: a review. Int J Nanomed. 2014;9:1.
Watkins R, Wu L, Zhang C, Davis RM, Xu B. Natural product-based nanomedicine: recent advances and issues. Int J Nanomed. 2015;10:6055.
Martinho N, Damgé C, Reis CP. Recent advances in drug delivery systems. J Biomater Nanobiotechnol. 2011;2:510.
Jahangirian H, Lemraski EG, Webster TJ, Rafiee-Moghaddam R, Abdollahi Y. A review of drug delivery systems based on nanotechnology and green chemistry: green nanomedicine. Int J Nanomed. 2017;12:2957.
Liu Z, Tabakman S, Welsher K, Dai H. Carbon nanotubes in biology and medicine: in vitro and in vivo detection, imaging and drug delivery. Nano Res. 2009;2:85–120.
Article CAS PubMed PubMed Central Google Scholar
Orive G, Gascon AR, Hernández RM, Domı́nguez-Gil A, Pedraz JL. Techniques: new approaches to the delivery of biopharmaceuticals. Trends Pharmacol Sci. 2004;25:382–7.
Razzacki SZ, Thwar PK, Yang M, Ugaz VM, Burns MA. Integrated microsystems for controlled drug delivery. Adv Drug Deliv Rev. 2004;56:185–98.
Article PubMed CAS Google Scholar
Arayne MS, Sultana N, Qureshi F. nanoparticles in delivery of cardiovascular drugs. Pak J Pharm Sci. 2007;20:340–8.
CAS PubMed Google Scholar
Patra JK, Baek K-H. Green nanobiotechnology: factors affecting synthesis and characterization techniques. J Nanomater. 2014;2014:219.
Joseph RR, Venkatraman SS. Drug delivery to the eye: what benefits do nanocarriers offer? Nanomedicine. 2017;12:683–702.
Mirza AZ, Siddiqui FA. Nanomedicine and drug delivery: a mini review. Int Nano Lett. 2014;4:94.
Rudramurthy GR, Swamy MK, Sinniah UR, Ghasemzadeh A. Nanoparticles: alternatives against drug-resistant pathogenic microbes. Molecules. 2016;21:836.
Article CAS PubMed Central Google Scholar
Lam P-L, Wong W-Y, Bian Z, Chui C-H, Gambari R. Recent advances in green nanoparticulate systems for drug delivery: efficient delivery and safety concern. Nanomedicine. 2017;12:357–85.
Haba Y, Kojima C, Harada A, Ura T, Horinaka H, Kono K. Preparation of poly (ethylene glycol)-modified poly (amido amine) dendrimers encapsulating gold nanoparticles and their heat-generating ability. Langmuir. 2007;23:5243–6.
Shi X, Sun K, Baker JR Jr. Spontaneous formation of functionalized dendrimer-stabilized gold nanoparticles. J Phys Chem C. 2008;112:8251–8.
Park S-H, Oh S-G, Mun J-Y, Han S-S. Loading of gold nanoparticles inside the DPPC bilayers of liposome and their effects on membrane fluidities. Coll Surf B. 2006;48:112–8.
de Villiers MM, Aramwit P, Kwon GS. Nanotechnology in drug delivery. New York: Springer; 2008.
Kabanov AV, Lemieux P, Vinogradov S, Alakhov V. Pluronic ® block copolymers: novel functional molecules for gene therapy. Adv Drug Deliv Rev. 2002;54:223–33.
Wang N, Feng Y. Elaborating the role of natural products-induced autophagy in cancer treatment: achievements and artifacts in the state of the art. BioMed Res Int. 2015;2015:934207.
PubMed PubMed Central Google Scholar
Ouattara B, Simard RE, Holley RA. Piette GJ-P, Bégin A: Antibacterial activity of selected fatty acids and essential oils against six meat spoilage organisms. Int J Food Microbiol. 1997;37:155–62.
Sharma G, Raturi K, Dang S, Gupta S, Gabrani R. Combinatorial antimicrobial effect of curcumin with selected phytochemicals on Staphylococcus epidermidis . J Asian Nat Prod Res. 2014;16:535–41.
Abdelwahab SI, Sheikh BY, Taha MME, How CW, Abdullah R, Yagoub U, El-Sunousi R, Eid EE. Thymoquinone-loaded nanostructured lipid carriers: preparation, gastroprotection, in vitro toxicity, and pharmacokinetic properties after extravascular administration. Int J Nanomed. 2013;8:2163.
Krauel K, Pitaksuteepong T, Davies NM, Rades T. Entrapment of bioactive molecules in poly (alkylcyanoacrylate) nanoparticles. Am J Drug Deliv. 2004;2:251–9.
Tan Q, Liu W, Guo C, Zhai G. Preparation and evaluation of quercetin-loaded lecithin-chitosan nanoparticles for topical delivery. Int J Nanomed. 2011;6:1621.
Sanna V, Roggio AM, Siliani S, Piccinini M, Marceddu S, Mariani A, Sechi M. Development of novel cationic chitosan-and anionic alginate–coated poly ( d, l- lactide-co-glycolide) nanoparticles for controlled release and light protection of resveratrol. Int J Nanomed. 2012;7:5501.
Casettari L, Illum L. Chitosan in nasal delivery systems for therapeutic drugs. J Control Release. 2014;190:189–200.
Obeid MA, Al Qaraghuli MM, Alsaadi M, Alzahrani AR, Niwasabutra K, Ferro VA. Delivering natural products and biotherapeutics to improve drug efficacy. Ther Deliv. 2017;8:947–56.
Miele E, Spinelli GP, Miele E, Di Fabrizio E, Ferretti E, Tomao S, Gulino A. Nanoparticle-based delivery of small interfering RNA: challenges for cancer therapy. Int J Nanomed. 2012;7:3637.
McNamara K, Tofail SA. Nanosystems: the use of nanoalloys, metallic, bimetallic, and magnetic nanoparticles in biomedical applications. Phys Chem Chem Phys. 2015;17:27981–95.
Saadeh Y, Vyas D. Nanorobotic applications in medicine: current proposals and designs. Am J Robot Surg. 2014;1:4–11.
Article PubMed PubMed Central Google Scholar
Oliveira ON Jr, Iost RM, Siqueira JR Jr, Crespilho FN, Caseli L. Nanomaterials for diagnosis: challenges and applications in smart devices based on molecular recognition. ACS Appl Mater Interfaces. 2014;6:14745–66.
De Jong WH, Borm PJ. Drug delivery and nanoparticles: applications and hazards. Int J Nanomed. 2008;3:133.
Article Google Scholar
Holzinger M, Le Goff A, Cosnier S. Nanomaterials for biosensing applications: a review. Front Chem. 2014;2:63.
Golovin YI, Gribanovsky SL, Golovin DY, Klyachko NL, Majouga AG, Master AM, Sokolsky M, Kabanov AV. Towards nanomedicines of the future: remote magneto-mechanical actuation of nanomedicines by alternating magnetic fields. J Control Release. 2015;219:43–60.
Lu H, Wang J, Wang T, Zhong J, Bao Y, Hao H. Recent progress on nanostructures for drug delivery applications. J Nanomater. 2016;2016:20.
Blanco E, Shen H, Ferrari M. Principles of nanoparticle design for overcoming biological barriers to drug delivery. Nat Biotechnol. 2015;33:941.
Kumari A, Kumar V, Yadav S. Nanotechnology: a tool to enhance therapeutic values of natural plant products. Trends Med Res. 2012;7:34–42.
Chen F, Ehlerding EB, Cai W. Theranostic nanoparticles. J Nucl Med. 2014;55:1919–22.
Swierczewska M, Han H, Kim K, Park J, Lee S. Polysaccharide-based nanoparticles for theranostic nanomedicine. Adv Drug Deliv Rev. 2016;99:70–84.
Chen K, Chen X. Design and development of molecular imaging probes. Curr Top Med Chem. 2010;10:1227–36.
Yhee JY, Son S, Kim SH, Park K, Choi K, Kwon IC. Self-assembled glycol chitosan nanoparticles for disease-specific theranostics. J Control Release. 2014;193:202–13.
Lee C-M, Jang D, Kim J, Cheong S-J, Kim E-M, Jeong M-H, Kim S-H, Kim DW, Lim ST, Sohn M-H, et al. Oleyl-Chitosan nanoparticles based on a dual probe for Optical/MR imaging in vivo. Bioconjug Chem. 2011;22:186–92.
Yang S-J, Lin F-H, Tsai H-M, Lin C-F, Chin H-C, Wong J-M, Shieh M-J. Alginate-folic acid-modified chitosan nanoparticles for photodynamic detection of intestinal neoplasms. Biomaterials. 2011;32:2174–82.
Ryu JH, Na JH, Ko HK, You DG, Park S, Jun E, Yeom HJ, Seo DH, Park JH, Jeong SY. Non-invasive optical imaging of cathepsin B with activatable fluorogenic nanoprobes in various metastatic models. Biomaterials. 2014;35:2302–11.
Lapčík L, Lapcik L, De Smedt S, Demeester J, Chabrecek P. Hyaluronan: preparation, structure, properties, and applications. Chem Rev. 1998;98:2663–84.
Article PubMed Google Scholar
Kim H, Kim Y, Kim I-H, Kim K, Choi Y. ROS-responsive activatable photosensitizing agent for imaging and photodynamic therapy of activated macrophages. Theranostics. 2014;4:1.
Choi KY, Chung H, Min KH, Yoon HY, Kim K, Park JH, Kwon IC, Jeong SY. Self-assembled hyaluronic acid nanoparticles for active tumor targeting. Biomaterials. 2010;31:106–14.
Kamat M, El-Boubbou K, Zhu DC, Lansdell T, Lu X, Li W, Huang X. Hyaluronic acid immobilized magnetic nanoparticles for active targeting and imaging of macrophages. Bioconjug Chem. 2010;21:2128–35.
Arpicco S, Lerda C, Dalla Pozza E, Costanzo C, Tsapis N, Stella B, Donadelli M, Dando I, Fattal E, Cattel L. Hyaluronic acid-coated liposomes for active targeting of gemcitabine. Eur J Pharm Biopharm. 2013;85:373–80.
Wang G, Gao S, Tian R, Miller-Kleinhenz J, Qin Z, Liu T, Li L, Zhang F, Ma Q, Zhu L. Theranostic hyaluronic acid-iron micellar nanoparticles for magnetic-field-enhanced in vivo cancer chemotherapy. ChemMedChem. 2018;13:78–86.
Choi KY, Jeon EJ, Yoon HY, Lee BS, Na JH, Min KH, Kim SY, Myung S-J, Lee S, Chen X. Theranostic nanoparticles based on PEGylated hyaluronic acid for the diagnosis, therapy and monitoring of colon cancer. Biomaterials. 2012;33:6186–93.
Gombotz WR, Wee S. Protein release from alginate matrices. Adv Drug Deliv Rev. 1998;31:267–85.
Lee KY, Mooney DJ. Alginate: properties and biomedical applications. Prog Polym Sci. 2012;37:106–26.
Baghbani F, Moztarzadeh F, Mohandesi JA, Yazdian F, Mokhtari-Dizaji M. Novel alginate-stabilized doxorubicin-loaded nanodroplets for ultrasounic theranosis of breast cancer. Int J Biol Macromol. 2016;93:512–9.
Podgórna K, Szczepanowicz K, Piotrowski M, Gajdošová M, Štěpánek F, Warszyński P. Gadolinium alginate nanogels for theranostic applications. Coll Surf B. 2017;153:183–9.
Moscovici M. Present and future medical applications of microbial exopolysaccharides. Front Microbiol. 1012;2015:6.
Ding Z, Liu P, Hu D, Sheng Z, Yi H, Gao G, Wu Y, Zhang P, Ling S, Cai L. Redox-responsive dextran based theranostic nanoparticles for near-infrared/magnetic resonance imaging and magnetically targeted photodynamic therapy. Biomater Sci. 2017;5:762–71.
Hong S-P, Kang SH, Kim DK, Kang BS. Paramagnetic nanoparticle-based targeting theranostic agent for c6 rat glioma cell. J Nanomater. 2016; 2016:7617894. https://doi.org/10.1155/2016/7617894 .
Mignani S, El Kazzouli S, Bousmina M, Majoral JP. Expand classical drug administration ways by emerging routes using dendrimer drug delivery systems: a concise overview. Adv Drug Deliv Rev. 2013;65:1316–30.
Lounnas V, Ritschel T, Kelder J, McGuire R, Bywater RP, Foloppe N. Current progress in structure-based rational drug design marks a new mindset in drug discovery. Comput Struc Biotechnol J. 2013;5:e201302011.
Mavromoustakos T, Durdagi S, Koukoulitsa C, Simcic M, Papadopoulos M, Hodoscek M, Golic Grdadolnik S. Strategies in the rational drug design. Curr Med Chem. 2011;18:2517–30.
Wong PT, Choi SK. Mechanisms of drug release in nanotherapeutic delivery systems. Chem Rev. 2015;115:3388–432.
Prachayasittikul V, Worachartcheewan A, Shoombuatong W, Songtawee N, Simeon S, Prachayasittikul V, Nantasenamat C. Computer-aided drug design of bioactive natural products. Curr Top Med Chem. 2015;15:1780–800.
Chen G, Roy I, Yang C, Prasad PN. Nanochemistry and nanomedicine for nanoparticle-based diagnostics and therapy. Chem Rev. 2016;116:2826–85.
Pelaz B, Alexiou C, Alvarez-Puebla RA, Alves F, Andrews AM, Ashraf S, Balogh LP, Ballerini L, Bestetti A, Brendel C, Bosi S. Diverse applications of nanomedicine. Acs Nano. 2017;11:2313–81.
Mattos BD, Rojas OJ, Magalhaes WLE. Biogenic silica nanoparticles loaded with neem bark extract as green, slow-release biocide. J Clean Prod. 2017;142:4206–13.
Kinnear C, Moore TL, Rodriguez-Lorenzo L, Rothen-Rutishauser B, Petri-Fink A. Form follows function: nanoparticle shape and its implications for nanomedicine. Chem Rev. 2017;117:11476–521.
Sethi M, Sukumar R, Karve S, Werner ME, Wang EC, Moore DT, Kowalczyk SR, Zhang L, Wang AZ. Effect of drug release kinetics on nanoparticle therapeutic efficacy and toxicity. Nanoscale. 2014;6:2321–7.
Mattos BD, Tardy BL, Magalhaes WLE, Rojas OJ. Controlled release for crop and wood protection: recent progress toward sustainable and safe nanostructured biocidal systems. J Control Release. 2017;262:139–50.
Siepmann F, Herrmann S, Winter G, Siepmann J. A novel mathematical model quantifying drug release from lipid implants. J Control Release. 2008;128:233–40.
Ding CZ, Li ZB. A review of drug release mechanisms from nanocarrier systems. Mater Sci Eng. 2017;76:1440–53.
Lee JH, Yeo Y. Controlled drug release from pharmaceutical nanocarriers. Chem Eng Sci. 2015;125:75–84.
Kamaly N, Yameen B, Wu J, Farokhzad OC. Degradable controlled-release polymers and polymeric nanoparticles: mechanisms of controlling drug release. Chem Rev. 2016;116:2602–63.
Torchilin VP. Multifunctional nanocarriers. Adv Drug Deliv Rev. 2012;64:302–15.
Pelaz B, del Pino P, Maffre P, Hartmann R, Gallego M, Rivera-Fernandez S, de la Fuente JM, Nienhaus GU, Parak WJ. Surface functionalization of nanoparticles with polyethylene glycol: effects on protein adsorption and cellular uptake. Acs Nano. 2015;9:6996–7008.
Almalik A, Benabdelkamel H, Masood A, Alanazi IO, Alradwan I, Majrashi MA, Alfadda AA, Alghamdi WM, Alrabiah H, Tirelli N, Alhasan AH. Hyaluronic acid coated chitosan nanoparticles reduced the immunogenicity of the formed protein corona. Sci Rep. 2017;7:10542.
Martens TF, Remaut K, Deschout H, Engbersen JFJ, Hennink WE, van Steenbergen MJ, Demeester J, De Smedt SC, Braeckmans K. Coating nanocarriers with hyaluronic acid facilitates intravitreal drug delivery for retinal gene therapy. J Control Release. 2015;202:83–92.
Kolhar P, Anselmo AC, Gupta V, Pant K, Prabhakarpandian B, Ruoslahti E, Mitragotri S. Using shape effects to target antibody-coated nanoparticles to lung and brain endothelium. Proc Natl Acad Sci USA. 2013;110:10753–8.
Gao WW, Zhang LF. Coating nanoparticles with cell membranes for targeted drug delivery. J Drug Target. 2015;23:619–26.
Muller J, Bauer KN, Prozeller D, Simon J, Mailander V, Wurm FR, Winzen S, Landfester K. Coating nanoparticles with tunable surfactants facilitates control over the protein corona. Biomaterials. 2017;115:1–8.
Gao H, Yang Z, Zhang S, Cao S, Shen S, Pang Z, Jiang X. Ligand modified nanoparticles increases cell uptake, alters endocytosis and elevates glioma distribution and internalization. Sci Rep. 2013;3:2534.
Jain A, Jain SK. Ligand-appended BBB-targeted nanocarriers (LABTNs). Crit Rev Ther Drug Carrier Syst. 2015;32:149–80.
Shen HX, Shi SJ, Zhang ZR, Gong T, Sun X. Coating solid lipid nanoparticles with hyaluronic acid enhances antitumor activity against melanoma stem-like cells. Theranostics. 2015;5:755–71.
Gao X, Zhang J, Xu Q, Huang Z, Wang YY, Shen Q. Hyaluronic acid-coated cationic nanostructured lipid carriers for oral vincristine sulfate delivery. Drug Dev Ind Pharm. 2017;43:661–7.
Wang T, Hou JH, Su C, Zhao L, Shi YJ. Hyaluronic acid-coated chitosan nanoparticles induce ROS-mediated tumor cell apoptosis and enhance antitumor efficiency by targeted drug delivery via CD44. J Nanobiotechnol. 2017;15:7.
Muro S. Challenges in design and characterization of ligand-targeted drug delivery systems. J Control Release. 2012;164:125–37.
Kou L, Sun J, Zhai Y, He Z. The endocytosis and intracellular fate of nanomedicines: implication for rational design. Asian J Pharm Sci. 2013;8:1–10.
Li Z, Zhang Y, Zhu D, Li S, Yu X, Zhao Y, Ouyang X, Xie Z, Li L. Transporting carriers for intracellular targeting delivery via non-endocytic uptake pathways. Drug delivery. 2017;24:45–55.
Salatin S, Yari Khosroushahi A. Overviews on the cellular uptake mechanism of polysaccharide colloidal nanoparticles. J Cell Mol Med. 2017;21:1668–86.
Anirudhan TS, Nair AS. Temperature and ultrasound sensitive gatekeepers for the controlled release of chemotherapeutic drugs from mesoporous silica nanoparticles. J Mater Chem B. 2018;6:428–39.
Al-Ahmady Z, Kostarelos K. Chemical components for the design of temperature-responsive vesicles as cancer therapeutics. Chem Rev. 2016;116:3883–918.
Bai Y, Xie FY, Tian W. Controlled self-assembly of thermo-responsive amphiphilic h-shaped polymer for adjustable drug release. Chin J Polym Sci. 2018;36:406–16.
Zhang Z, Zhang D, Wei L, Wang X, Xu YL, Li HW, Ma M, Chen B, Xiao LH. Temperature responsive fluorescent polymer nanoparticles (TRFNPs) for cellular imaging and controlled releasing of drug to living cells. Coll Surf B. 2017;159:905–12.
Guo Y, Zhang Y, Ma J, Li Q, Li Y, Zhou X, Zhao D, Song H, Chen Q, Zhu X. Light/magnetic hyperthermia triggered drug released from multi-functional thermo-sensitive magnetoliposomes for precise cancer synergetic theranostics. J Control Release. 2017;272:145–58.
Hervault A, Thanh NT. Magnetic nanoparticle-based therapeutic agents for thermo-chemotherapy treatment of cancer. Nanoscale. 2014;6:11553–73.
Mathiyazhakan M, Wiraja C, Xu CJ: A Concise Review of Gold Nanoparticles-Based Photo-Responsive Liposomes for Controlled Drug Delivery. Nano - Micro Letters 2018, 10.
Xu L, Qiu LZ, Sheng Y, Sun YX, Deng LH, Li XQ, Bradley M, Zhang R. Biodegradable pH-responsive hydrogels for controlled dual-drug release. J Mater Chem B. 2018;6:510–7.
Ma GL, Lin WF, Yuan ZF, Wu J, Qian HF, Xua LB, Chen SF. Development of ionic strength/pH/enzyme triple-responsive zwitterionic hydrogel of the mixed l -glutamic acid and l -lysine polypeptide for site-specific drug delivery. J Mater Chem B. 2017;5:935–43.
Grillo R, Gallo J, Stroppa DG, Carbo-Argibay E, Lima R, Fraceto LF, Banobre-Lopez M. Sub-micrometer magnetic nanocomposites: insights into the effect of magnetic nanoparticles interactions on the optimization of SAR and MRI performance. Acs Appl Mater Interfaces. 2016;8:25777–87.
Alonso J, Khurshid H, Devkota J, Nemati Z, Khadka NK, Srikanth H, Pan JJ, Phan MH. Superparamagnetic nanoparticles encapsulated in lipid vesicles for advanced magnetic hyperthermia and biodetection. J Appl Phys. 2016;119:083904.
Ulbrich K, Hola K, Subr V, Bakandritsos A, Tucek J, Zboril R. Targeted drug delivery with polymers and magnetic nanoparticles: covalent and noncovalent approaches, release control, and clinical studies. Chem Rev. 2016;116:5338–431.
Chen CW, Syu WJ, Huang TC, Lee YC, Hsiao JK, Huang KY, Yu HP, Liao MY, Lai PS. Encapsulation of Au/Fe 3 O 4 nanoparticles into a polymer nanoarchitecture with combined near infrared-triggered chemo-photothermal therapy based on intracellular secondary protein understanding. J Mater Chem B. 2017;5:5774–82.
Portero A, Remunan-Lopez C, Criado M, Alonso M. Reacetylated chitosan microspheres for controlled delivery of anti-microbial agents to the gastric mucosa. J Microencapsul. 2002;19:797–809.
Artursson P, Lindmark T, Davis SS, Illum L. Effect of chitosan on the permeability of monolayers of intestinal epithelial cells (Caco-2). Pharm Res. 1994;11:1358–61.
Fernández-Urrusuno R, Calvo P, Remuñán-López C, Vila-Jato JL, Alonso MJ. Enhancement of nasal absorption of insulin using chitosan nanoparticles. Pharm Res. 1999;16:1576–81.
De Campos AM, Sánchez A, Alonso MJ. Chitosan nanoparticles: a new vehicle for the improvement of the delivery of drugs to the ocular surface. Application to cyclosporin A. Int J Pharm. 2001;224:159–68.
Al-Qadi S, Grenha A, Carrión-Recio D, Seijo B, Remuñán-López C. Microencapsulated chitosan nanoparticles for pulmonary protein delivery: in vivo evaluation of insulin-loaded formulations. J Control Release. 2012;157:383–90.
Silva MM, Calado R, Marto J, Bettencourt A, Almeida AJ, Gonçalves L. Chitosan Nanoparticles as a mucoadhesive drug delivery system for ocular administration. Mar Drugs. 2017;15:370.
Article PubMed Central Google Scholar
Pistone S, Goycoolea FM, Young A, Smistad G, Hiorth M. Formulation of polysaccharide-based nanoparticles for local administration into the oral cavity. Eur J Pharm Sci. 2017;96:381–9.
Liu S, Yang S, Ho PC. Intranasal administration of carbamazepine-loaded carboxymethyl chitosan nanoparticles for drug delivery to the brain. Asian J Pharm Sci. 2018;13:72–81.
Jain A, Jain SK. Optimization of chitosan nanoparticles for colon tumors using experimental design methodology. Artif Cells Nanomed Biotechnol. 2016;44:1917–26.
Sosnik A. Alginate particles as platform for drug delivery by the oral route: state-of-the-art. ISRN Pharm. 2014;2014:926157.
Patil NH, Devarajan PV. Insulin-loaded alginic acid nanoparticles for sublingual delivery. Drug Deliv. 2016;23:429–36.
Haque S, Md S, Sahni JK, Ali J, Baboota S. Development and evaluation of brain targeted intranasal alginate nanoparticles for treatment of depression. J Psychiatr Res. 2014;48:1–12.
Román JV, Galán MA, del Valle EMM. Preparation and preliminary evaluation of alginate crosslinked microcapsules as potential drug delivery system (DDS) for human lung cancer therapy. Biomed Phys Eng Expr. 2016;2:035015.
Garrait G, Beyssac E, Subirade M. Development of a novel drug delivery system: chitosan nanoparticles entrapped in alginate microparticles. J Microencapsul. 2014;31:363–72.
Costa J, Silva N, Sarmento B, Pintado M. Potential chitosan-coated alginate nanoparticles for ocular delivery of daptomycin. Eur J Clin Microbiol Infect Dis. 2015;34:1255–62.
Goswami S, Naik S. Natural gums and its pharmaceutical application. J Sci Innovative Res. 2014;3:112–21.
Laffleur F, Michalek M. Modified xanthan gum for buccal delivery—a promising approach in treating sialorrhea. Int J Biol Macromol. 2017;102:1250–6.
Huang J, Deng Y, Ren J, Chen G, Wang G, Wang F, Wu X. Novel in situ forming hydrogel based on xanthan and chitosan re-gelifying in liquids for local drug delivery. Carbohydr Polym. 2018;186:54–63.
Menzel C, Jelkmann M, Laffleur F, Bernkop-Schnürch A. Nasal drug delivery: design of a novel mucoadhesive and in situ gelling polymer. Int J Pharm. 2017;517:196–202.
Sun B, Zhang M, Shen J, He Z, Fatehi P, Ni Y. Applications of cellulose-based materials in sustained drug delivery systems. Curr Med Chem. 2017. https://doi.org/10.2174/0929867324666170705143308 .
Elseoud WSA, Hassan ML, Sabaa MW, Basha M, Hassan EA, Fadel SM. Chitosan nanoparticles/cellulose nanocrystals nanocomposites as a carrier system for the controlled release of repaglinide. Int J Biol Macromol. 2018;111:604–13.
Agarwal T, Narayana SGH, Pal K, Pramanik K, Giri S, Banerjee I. Calcium alginate-carboxymethyl cellulose beads for colon-targeted drug delivery. Int J Biol Macromol. 2015;75:409–17.
Hansen K, Kim G, Desai KG, Patel H, Olsen KF, Curtis-Fisk J, Tocce E, Jordan S, Schwendeman SP. Feasibility investigation of cellulose polymers for mucoadhesive nasal drug delivery applications. Mol Pharm. 2015;12:2732–41.
Bozzuto G, Molinari A. Liposomes as nanomedical devices. Int J Nanomed. 2015;10:975.
Sercombe L, Veerati T, Moheimani F, Wu SY, Sood AK, Hua S. Advances and challenges of liposome assisted drug delivery. Front Pharm. 2015;6:286.
Kotla NG, Chandrasekar B, Rooney P, Sivaraman G, Larrañaga A, Krishna KV, Pandit A, Rochev Y. Biomimetic lipid-based nanosystems for enhanced dermal delivery of drugs and bioactive agents. ACS Biomater Sci Eng. 2017;3:1262–72.
Akbarzadeh A, Rezaei-Sadabady R, Davaran S, Joo SW, Zarghami N, Hanifehpour Y, Samiei M, Kouhi M, Nejati-Koshki K. Liposome: classification, preparation, and applications. Nanoscale Res Lett. 2013;8:102.
Mohan A, Narayanan S, Sethuraman S, Krishnan UM. Novel resveratrol and 5-fluorouracil coencapsulated in PEGylated nanoliposomes improve chemotherapeutic efficacy of combination against head and neck squamous cell carcinoma. BioMed res int. 2014;2014:424239.
Dimov N, Kastner E, Hussain M, Perrie Y, Szita N. Formation and purification of tailored liposomes for drug delivery using a module-based micro continuous-flow system. Sci Rep. 2017;7:12045.
Zylberberg C, Matosevic S. Pharmaceutical liposomal drug delivery: a review of new delivery systems and a look at the regulatory landscape. Drug Deliv. 2016;23:3319–29.
Sapsford KE, Algar WR, Berti L, Gemmill KB, Casey BJ, Oh E, Stewart MH, Medintz IL. Functionalizing nanoparticles with biological molecules: developing chemistries that facilitate nanotechnology. Chem Rev. 2013;113:1904–2074.
Zhang L, Gu F, Chan J, Wang A, Langer R, Farokhzad O. Nanoparticles in medicine: therapeutic applications and developments. Clin Pharmacol Ther. 2008;83:761–9.
Miyata K, Christie RJ, Kataoka K. Polymeric micelles for nano-scale drug delivery. React Funct Polym. 2011;71:227–34.
Xu W, Ling P, Zhang T. Polymeric micelles, a promising drug delivery system to enhance bioavailability of poorly water-soluble drugs. J Drug Deliv. 2013;2013:340315.
Kulthe SS, Choudhari YM, Inamdar NN, Mourya V. Polymeric micelles: authoritative aspects for drug delivery. Design Monomers Polym. 2012;15:465–521.
Devarajan PV, Jain S. Targeted drug delivery: concepts and design. Berlin: Springer; 2016.
Mourya V, Inamdar N, Nawale R, Kulthe S. Polymeric micelles: general considerations and their applications. Ind J Pharm Educ Res. 2011;45:128–38.
Wakaskar RR. Polymeric micelles for drug delivery. Int J Drug Dev Res. 2017;9:1–2.
Mandal A, Bisht R, Rupenthal ID, Mitra AK. Polymeric micelles for ocular drug delivery: from structural frameworks to recent preclinical studies. J Control Release. 2017;248:96–116.
Li Q, Lai KL, Chan PS, Leung SC, Li HY, Fang Y, To KK, Choi CHJ, Gao QY, Lee TW. Micellar delivery of dasatinib for the inhibition of pathologic cellular processes of the retinal pigment epithelium. Coll Surf B. 2016;140:278–86.
Kesharwani P, Xie L, Banerjee S, Mao G, Padhye S, Sarkar FH, Iyer AK. Hyaluronic acid-conjugated polyamidoamine dendrimers for targeted delivery of 3, 4-difluorobenzylidene curcumin to CD44 overexpressing pancreatic cancer cells. Coll Surf B. 2015;136:413–23.
Zhu J, Shi X. Dendrimer-based nanodevices for targeted drug delivery applications. J Mater Chem B. 2013;1:4199–211.
Madaan K, Kumar S, Poonia N, Lather V, Pandita D. Dendrimers in drug delivery and targeting: drug-dendrimer interactions and toxicity issues. J Pharm Bioallied Sci. 2014;6:139.
Cheng Y, Xu Z, Ma M, Xu T. Dendrimers as drug carriers: applications in different routes of drug administration. J Pharm Sci. 2008;97:123–43.
Noriega-Luna B, Godínez LA, Rodríguez FJ, Rodríguez A, Larrea G, Sosa-Ferreyra C, Mercado-Curiel R, Manríquez J, Bustos E. Applications of dendrimers in drug delivery agents, diagnosis, therapy, and detection. J Nanomater. 2014;2014:39.
Tripathy S, Das M. Dendrimers and their applications as novel drug delivery carriers. J Appl Pharm Sci. 2013;3:142–9.
Kesharwani P, Jain K, Jain NK. Dendrimer as nanocarrier for drug delivery. Progr Polym Sci. 2014;39:268–307.
Jain K, Gupta U, Jain NK. Dendronized nanoconjugates of lysine and folate for treatment of cancer. Eur J Pharm Biopharm. 2014;87:500–9.
Kaur A, Jain K, Mehra NK, Jain N. Development and characterization of surface engineered PPI dendrimers for targeted drug delivery. Artif Cells Nanomed Biotechnol. 2017;45:414–25.
Choi S-J, Lee JK, Jeong J, Choy J-H. Toxicity evaluation of inorganic nanoparticles: considerations and challenges. Mol Cell Toxicol. 2013;9:205–10.
Kong F-Y, Zhang J-W, Li R-F, Wang Z-X, Wang W-J, Wang W. Unique roles of gold nanoparticles in drug delivery, targeting and imaging applications. Molecules. 2017;22:1445.
Prusty K, Swain SK. Nano silver decorated polyacrylamide/dextran nanohydrogels hybrid composites for drug delivery applications. Mater Sci Eng. 2018;85:130–41.
Marcu A, Pop S, Dumitrache F, Mocanu M, Niculite C, Gherghiceanu M, Lungu C, Fleaca C, Ianchis R, Barbut A. Magnetic iron oxide nanoparticles as drug delivery system in breast cancer. Appl Surf Sci. 2013;281:60–5.
Junyaprasert VB, Morakul B. Nanocrystals for enhancement of oral bioavailability of poorly water-soluble drugs. Asian J Pharm Sci. 2015;10:13–23.
Du J, Li X, Zhao H, Zhou Y, Wang L, Tian S, Wang Y. Nanosuspensions of poorly water-soluble drugs prepared by bottom-up technologies. Int J Pharm. 2015;495:738–49.
Ni R, Zhao J, Liu Q, Liang Z, Muenster U, Mao S. Nanocrystals embedded in chitosan-based respirable swellable microparticles as dry powder for sustained pulmonary drug delivery. Eur J Pharm Sci. 2017;99:137–46.
McNamara K, Tofail SA. Nanoparticles in biomedical applications. Adv Phys. 2017;2:54–88.
Kudr J, Haddad Y, Richtera L, Heger Z, Cernak M, Adam V, Zitka O. Magnetic nanoparticles: from design and synthesis to real world applications. Nanomaterials. 2017;7:243.
Article PubMed Central CAS Google Scholar
Prasad PN. Nanophotonics. New York: Wiley; 2004.
Book Google Scholar
Volkov Y. Quantum dots in nanomedicine: recent trends, advances and unresolved issues. Biochem Biophys Res Commun. 2015;468:419–27.
Liu J, Lau SK, Varma VA, Moffitt RA, Caldwell M, Liu T, Young AN, Petros JA, Osunkoya AO, Krogstad T. Molecular mapping of tumor heterogeneity on clinical tissue specimens with multiplexed quantum dots. ACS Nano. 2010;4:2755–65.
Xu G, Zeng S, Zhang B, Swihart MT, Yong K-T, Prasad PN. New generation cadmium-free quantum dots for biophotonics and nanomedicine. Chem Rev. 2016;116:12234–327.
Shi Y, Pramanik A, Tchounwou C, Pedraza F, Crouch RA, Chavva SR, Vangara A, Sinha SS, Jones S, Sardar D. Multifunctional biocompatible graphene oxide quantum dots decorated magnetic nanoplatform for efficient capture and two-photon imaging of rare tumor cells. ACS Appl Mater Interfaces. 2015;7:10935–43.
Han H-S, Niemeyer E, Huang Y, Kamoun WS, Martin JD, Bhaumik J, Chen Y, Roberge S, Cui J, Martin MR. Quantum dot/antibody conjugates for in vivo cytometric imaging in mice. Proc Natl Acad Sci. 2015;112:1350–5.
So M-K, Xu C, Loening AM, Gambhir SS, Rao J. Self-illuminating quantum dot conjugates for in vivo imaging. Nat Biotechnol. 2006;24:339.
Zheng F-F, Zhang P-H, Xi Y, Chen J-J, Li L-L, Zhu J-J. Aptamer/graphene quantum dots nanocomposite capped fluorescent mesoporous silica nanoparticles for intracellular drug delivery and real-time monitoring of drug release. Anal Chem. 2015;87:11739–45.
Huang C-L, Huang C-C, Mai F-D, Yen C-L, Tzing S-H, Hsieh H-T, Ling Y-C, Chang J-Y. Application of paramagnetic graphene quantum dots as a platform for simultaneous dual-modality bioimaging and tumor-targeted drug delivery. J Mater Chem B. 2015;3:651–64.
Olerile LD, Liu Y, Zhang B, Wang T, Mu S, Zhang J, Selotlegeng L, Zhang N. Near-infrared mediated quantum dots and paclitaxel co-loaded nanostructured lipid carriers for cancer theragnostic. Coll Surf B. 2017;150:121–30.
Cai X, Luo Y, Zhang W, Du D, Lin Y. pH-Sensitive ZnO quantum dots–doxorubicin nanoparticles for lung cancer targeted drug delivery. ACS Appl Mater Interfaces. 2016;8:22442–50.
Balaji AB, Pakalapati H, Khalid M, Walvekar R, Siddiqui H. Natural and synthetic biocompatible and biodegradable polymers. In: Shimpi NG (ed) Biodegradable and biocompatible polymer composites: processing, properties and applications. Woodhead Publishing series in composites science and engineering. Duxford: Woodhead Publishing; 2017. p. 3–32.
Bassas-Galia M, Follonier S, Pusnik M, Zinn M. Natural polymers: a source of inspiration. In: Bioresorbable polymers for biomedical applications. New York: Elsevier; 2017. p. 31–64.
Chapter Google Scholar
Lohcharoenkal W, Wang L, Chen YC, Rojanasakul Y. Protein nanoparticles as drug delivery carriers for cancer therapy. BioMed Res Int. 2014;2014:180549.
Liu Z, Jiao Y, Wang Y, Zhou C, Zhang Z. Polysaccharides-based nanoparticles as drug delivery systems. Adv Drug Deliv Rev. 2008;60:1650–62.
Poole-Warren L, Patton A. Introduction to biomedical polymers and biocompatibility. In: Biosynthetic polymers for medical applications. New York: Elsevier; 2016. p. 3–31.
Pertici G. Introduction to bioresorbable polymers for biomedical applications. In: Biosynthetic polymers for medical applications. New York: Elsevier; 2016. p. 3–29.
Cardoso MJ, Costa RR, Mano JF. Marine origin polysaccharides in drug delivery systems. Mar Drugs. 2016;14:34.
Yu Z, Yu M, Zhang Z, Hong G, Xiong Q. Bovine serum albumin nanoparticles as controlled release carrier for local drug delivery to the inner ear. Nanoscale Res Lett. 2014;9:343.
Robinson M, Zhang X. The world medicines situation. Traditional medicines: global situation, issues and challenges. Geneva: World Health Organization; 2011. p. 1–12.
Atanasov AG, Waltenberger B, Pferschy-Wenzig E-M, Linder T, Wawrosch C, Uhrin P, Temml V, Wang L, Schwaiger S, Heiss EH. Discovery and resupply of pharmacologically active plant-derived natural products: a review. Biotechnol Adv. 2015;33:1582–614.
David B, Wolfender J-L, Dias DA. The pharmaceutical industry and natural products: historical status and new trends. Phytochem Rev. 2015;14:299–315.
Namdari M, Eatemadi A, Soleimaninejad M, Hammed AT. A brief review on the application of nanoparticle enclosed herbal medicine for the treatment of infective endocarditis. Biomed Pharm. 2017;87:321–31.
Heinrich M. Ethnopharmacology in the 21st century-grand challenges. Front Pharm. 2010;1:8.
Kinghorn AD, Pan L, Fletcher JN, Chai H. The relevance of higher plants in lead compound discovery programs. J Nat Prod. 2011;74:1539–55.
Yuan H, Ma Q, Ye L, Piao G. The traditional medicine and modern medicine from natural products. Molecules. 2016;21:559.
Patra JK, Das G, Baek K-H. Towards a greener environment: synthesis and applications of green nanoparticles. Pak J Agric Sci. 2016;53:59–79.
Duncan R, Gaspar R. Nanomedicine (s) under the microscope. Mol Pharm. 2011;8:2101–41.
Ramana KV, Singhal SS, Reddy AB. Therapeutic potential of natural pharmacological agents in the treatment of human diseases. BioMed Res Int. 2014;2014:573452.
Guo W. Green technology for nanoparticles in biomedical applications. In: Rai M, Posten C, editors. Green biosynthesis of nanoparticles: mechanisms and applications. Wallington: CABI; 2013.
Wicki A, Witzigmann D, Balasubramanian V, Huwyler J. Nanomedicine in cancer therapy: challenges, opportunities, and clinical applications. J Control Release. 2015;200:138–57.
Brigger I, Dubernet C, Couvreur P. Nanoparticles in cancer therapy and diagnosis. Adv Drug Deliv Rev. 2002;54:631–51.
Yohan D, Chithrani BD. Applications of nanoparticles in nanomedicine. J Biomed Nanotechnol. 2014;10:2371–92.
Ambesh P, Campia U, Obiagwu C, Bansal R, Shetty V, Hollander G, Shani J. Nanomedicine in coronary artery disease. Indian Heart J. 2017;69:244–51.
Grazu V, Moros M, Sánchez-Espinel C. Nanocarriers as nanomedicines: design concepts and recent advances. In: Frontiers of nanoscience. Vol. 4, New York: Elsevier; 2012. p. 337–440.
Rizzo LY, Theek B, Storm G, Kiessling F, Lammers T. Recent progress in nanomedicine: therapeutic, diagnostic and theranostic applications. Curr Opin Biotechnol. 2013;24:1159–66.
Devasena T. Diagnostic and therapeutic nanomaterials. In: Therapeutic and diagnostic nanomaterials. New York: Springer; 2017. p. 1–13.
Ventola CL. Progress in nanomedicine: approved and investigational nanodrugs. Pharm Ther. 2017;42:742.
Havel H, Finch G, Strode P, Wolfgang M, Zale S, Bobe I, Youssoufian H, Peterson M, Liu M. Nanomedicines: from bench to bedside and beyond. AAPS J. 2016;18:1373–8.
Kumar A, Chen F, Mozhi A, Zhang X, Zhao Y, Xue X, Hao Y, Zhang X, Wang PC, Liang X-J. Innovative pharmaceutical development based on unique properties of nanoscale delivery formulation. Nanoscale. 2013;5:8307–25.
Boroumand Moghaddam A, Namvar F, Moniri M, Md Tahir P, Azizi S, Mohamad R. Nanoparticles biosynthesized by fungi and yeast: a review of their preparation, properties, and medical applications. Molecules. 2015;20:16540–65.
Metz KM, Sanders SE, Pender JP, Dix MR, Hinds DT, Quinn SJ, Ward AD, Duffy P, Cullen RJ, Colavita PE. Green synthesis of metal nanoparticles via natural extracts: the biogenic nanoparticle corona and its effects on reactivity. ACS Sustain Chem Eng. 2015;3:1610–7.
Paul D, Sinha SN. Extracellular synthesis of silver nanoparticles using Pseudomonas aeruginosa KUPSB12 and its antibacterial activity. JJBS. 2014;7:245–50.
Kushwaha A, Singh VK, Bhartariya J, Singh P, Yasmeen K. Isolation and identification of E. coli bacteria for the synthesis of silver nanoparticles: characterization of the particles and study of antibacterial activity. Eur J Exp Biol. 2015;5:65–70.
Iravani S. Bacteria in nanoparticle synthesis: current status and future prospects. Int Sch Res Notices. 2014;2014:359316.
Mittal AK, Chisti Y, Banerjee UC. Synthesis of metallic nanoparticles using plant extracts. Biotechnol Adv. 2013;31:346–56.
Khan HA, Sakharkar MK, Nayak A, Kishore U, Khan A. 14-nanoparticles for biomedical applications: an overview. In: Narayan R, editor. Nanobiomaterials. Cambridge: Woodhead Publishing; 2018. p. 357–84.
Aravamudhan A, Ramos DM, Nada AA, Kumbar SG. Natural polymers: polysaccharides and their derivatives for biomedical applications. In: Natural and synthetic biomedical polymers. New York: Elsevier; 2014. p. 67–89.
Franci G, Falanga A, Galdiero S, Palomba L, Rai M, Morelli G, Galdiero M. Silver nanoparticles as potential antibacterial agents. Molecules. 2015;20:8856–74.
Pajardi G, Rapisarda V, Somalvico F, Scotti A, Russo GL, Ciancio F, Sgrò A, Nebuloni M, Allevi R, Torre ML. Skin substitutes based on allogenic fibroblasts or keratinocytes for chronic wounds not responding to conventional therapy: a retrospective observational study. Int Wound J. 2016;13:44–52.
Rahimi G, Alizadeh F, Khodavandi A. Mycosynthesis of silver nanoparticles from Candida albicans and its antibacterial activity against Escherichia coli and Staphylococcus aureus . Trop J Pharm Res. 2016;15:371–5.
Ali M, Kim B, Belfield KD, Norman D, Brennan M, Ali GS. Inhibition of Phytophthora parasitica and P. capsici by silver nanoparticles synthesized using aqueous extract of Artemisia absinthium . Phytopathology. 2015;105:1183–90.
Malapermal V, Botha I, Krishna SBN, Mbatha JN. Enhancing antidiabetic and antimicrobial performance of Ocimum basilicum , and Ocimum sanctum (L.) using silver nanoparticles. Saudi J Biol Sci. 2017;24:1294–305.
Sankar R, Karthik A, Prabu A, Karthik S, Shivashangari KS, Ravikumar V. Origanum vulgare mediated biosynthesis of silver nanoparticles for its antibacterial and anticancer activity. Coll Surf B. 2013;108:80–4.
Patra JK, Ali MS, Oh I-G, Baek K-H. Proteasome inhibitory, antioxidant, and synergistic antibacterial and anticandidal activity of green biosynthesized magnetic Fe3O4 nanoparticles using the aqueous extract of corn ( Zea mays L.) ear leaves. Artif Cells Nanomed Biotechnol. 2017;45:349–56.
Patra JK, Baek K-H. Antibacterial activity and synergistic antibacterial potential of biosynthesized silver nanoparticles against foodborne pathogenic bacteria along with its anticandidal and antioxidant effects. Front Microbiol. 2017;8:167.
Patra JK, Kwon Y, Baek K-H. Green biosynthesis of gold nanoparticles by onion peel extract: synthesis, characterization and biological activities. Adv Powder Technol. 2016;27:2204–13.
Patra JK, Baek K-H. Biosynthesis of silver nanoparticles using aqueous extract of silky hairs of corn and investigation of its antibacterial and anticandidal synergistic activity and antioxidant potential. IET Nanobiotechnol. 2016;10:326–33.
Patra JK, Baek K-H. Comparative study of proteasome inhibitory, synergistic antibacterial, synergistic anticandidal, and antioxidant activities of gold nanoparticles biosynthesized using fruit waste materials. Int J Nanomed. 2016;11:4691.
Patra JK, Baek K-H. Green synthesis of silver chloride nanoparticles using Prunus persica L. outer peel extract and investigation of antibacterial, anticandidal, antioxidant potential. Green Chem Lett Rev. 2016;9:132–42.
Patra JK, Das G, Baek K-H. Phyto-mediated biosynthesis of silver nanoparticles using the rind extract of watermelon ( Citrullus lanatus ) under photo-catalyzed condition and investigation of its antibacterial, anticandidal and antioxidant efficacy. J Photochem Photobiol B. 2016;161:200–10.
Wilczewska AZ, Niemirowicz K, Markiewicz KH, Car H. Nanoparticles as drug delivery systems. Pharmacol Rep. 2012;64:1020–37.
Zhu Z, Li Y, Yang X, Pan W, Pan H. The reversion of anti-cancer drug antagonism of tamoxifen and docetaxel by the hyaluronic acid-decorated polymeric nanoparticles. Pharmacol Res. 2017;126:84–96.
Dias DA, Urban S, Roessner U. A historical overview of natural products in drug discovery. Metabolites. 2012;2:303–36.
Gupta U, Sharma S, Khan I, Gothwal A, Sharma AK, Singh Y, Chourasia MK, Kumar V. Enhanced apoptotic and anticancer potential of paclitaxel loaded biodegradable nanoparticles based on chitosan. Int J Biol Macromol. 2017;98:810–9.
Chang C-H, Huang W-Y, Lai C-H, Hsu Y-M, Yao Y-H, Chen T-Y, Wu J-Y, Peng S-F, Lin Y-H. Development of novel nanoparticles shelled with heparin for berberine delivery to treat Helicobacter pylori. Acta Biomaterialia. 2011;7:593–603.
Aldawsari HM, Hosny KM. Solid lipid nanoparticles of Vancomycin loaded with Ellagic acid as a tool for overcoming nephrotoxic side effects: preparation, characterization, and nephrotoxicity evaluation. J Drug Deliv Sci Technol. 2018;45:76–80.
Dian L, Yu E, Chen X, Wen X, Zhang Z, Qin L, Wang Q, Li G, Wu C. Enhancing oral bioavailability of quercetin using novel soluplus polymeric micelles. Nanoscale Res Lett. 2014;9:684.
Spillmann CM, Naciri J, Algar WR, Medintz IL, Delehanty JB. Multifunctional liquid crystal nanoparticles for intracellular fluorescent imaging and drug delivery. ACS Nano. 2014;8:6986–97.
Purama RK, Goswami P, Khan AT, Goyal A. Structural analysis and properties of dextran produced by Leuconostoc mesenteroides NRRL B-640. Carbohydr Polym. 2009;76:30–5.
Agarwal A, Gupta U, Asthana A, Jain NK. Dextran conjugated dendritic nanoconstructs as potential vectors for anti-cancer agent. Biomaterials. 2009;30:3588–96.
Barenholz YC. Doxil ® —the first FDA-approved nano-drug: lessons learned. J Control Release. 2012;160:117–34.
Maeng JH, Lee D-H, Jung KH, Bae Y-H, Park I-S, Jeong S, Jeon Y-S, Shim C-K, Kim W, Kim J. Multifunctional doxorubicin loaded superparamagnetic iron oxide nanoparticles for chemotherapy and magnetic resonance imaging in liver cancer. Biomaterials. 2010;31:4995–5006.
Bonechi C, Martini S, Ciani L, Lamponi S, Rebmann H, Rossi C, Ristori S. Using liposomes as carriers for polyphenolic compounds: the case of trans-resveratrol. PLoS ONE. 2012;7:e41438.
Noorafshan A, Ashkani-Esfahani S. A review of therapeutic effects of curcumin. Curr Pharm Des. 2013;19:2032–46.
Wei X, Senanayake TH, Bohling A, Vinogradov SV. Targeted nanogel conjugate for improved stability and cellular permeability of curcumin: synthesis, pharmacokinetics, and tumor growth inhibition. Mol Pharm. 2014;11:3112–22.
Feng T, Wei Y, Lee RJ, Zhao L. Liposomal curcumin and its application in cancer. Int J Nanomed. 2017;12:6027.
Cheng C, Peng S, Li Z, Zou L, Liu W, Liu C. Improved bioavailability of curcumin in liposomes prepared using a pH-driven, organic solvent-free, easily scalable process. RSC Adv. 2017;7:25978–86.
Bilia AR, Guccione C, Isacchi B, Righeschi C, Firenzuoli F, Bergonzi MC. Essential oils loaded in nanosystems: a developing strategy for a successful therapeutic approach. Evid Based Complement Alternat Med. 2014;2014:651593.
Sainz V, Conniot J, Matos AI, Peres C, Zupanǒiǒ E, Moura L, Silva LC, Florindo HF, Gaspar RS. Regulatory aspects on nanomedicines. Biochem Biophys Res Commun. 2015;468:504–10.
Hassan S, Prakash G, Ozturk AB, Saghazadeh S, Sohail MF, Seo J, Dokmeci MR, Zhang YS, Khademhosseini A. Evolution and clinical translation of drug delivery nanomaterials. Nano Today. 2017;15:91–106.
Agrahari V, Agrahari V. Facilitating the translation of nanomedicines to a clinical product: challenges and opportunities. Drug Discov Today. 2018;23(5):974–91.
Caster JM, Patel AN, Zhang T, Wang A. Investigational nanomedicines in 2016: a review of nanotherapeutics currently undergoing clinical trials. Wiley Interdiscip Rev. 2016;2017:9.
Wacker MG, Proykova A, Santos GML. Dealing with nanosafety around the globe—regulation vs. innovation. Int J Pharm. 2016;509:95–106.
Lin P-C, Lin S, Wang PC, Sridhar R. Techniques for physicochemical characterization of nanomaterials. Biotechnol Adv. 2014;32:711–26.
Grossman JH, Crist RM, Clogston JD. Early development challenges for drug products containing nanomaterials. AAPS J. 2017;19:92–102.
Tinkle S, McNeil SE, Mühlebach S, Bawa R, Borchard G, Barenholz YC, Tamarkin L, Desai N. Nanomedicines: addressing the scientific and regulatory gap. Ann NY Acad Sci. 2014;1313:35–56.
Pandit A, Zeugolis DI. Twenty-five years of nano-bio-materials: have we revolutionized healthcare? Fut Med. 2016;11(9):985–7.
Bobo D, Robinson KJ, Islam J, Thurecht KJ, Corrie SR. Nanoparticle-based medicines: a review of FDA-approved materials and clinical trials to date. Pharm Res. 2016;33:2373–87.
Tran S, DeGiovanni P-J, Piel B, Rai P. Cancer nanomedicine: a review of recent success in drug delivery. Clin Transl Med. 2017;6:44.
Anselmo AC, Mitragotri S. Nanoparticles in the clinic. Bioeng Transl Med. 2016;1:10–29.
Grumezescu AM. Nanoscale fabrication, optimization, scale-up and biological aspects of pharmaceutical nanotechnology. New York: William Andrew; 2017.
Caster JM, Patel AN, Zhang T, Wang A. Investigational nanomedicines in 2016: a review of nanotherapeutics currently undergoing clinical trials. Wiley Interdiscip Rev. 2017;9:e1416.
Drug approvals and databases. https://www.fda.gov/Drugs/InformationOnDrugs/default.htm . Accessed 16 Aug 2018.
D’Mello SR, Cruz CN, Chen M-L, Kapoor M, Lee SL, Tyner KM. The evolving landscape of drug products containing nanomaterials in the United States. Nat Nanotechnol. 2017;12:523.
Download references
Authors’ contributions
JKP, GD, LFF, EVRC, MDPRT, LSAT, LADT, RG, MKS, SS and SH wrote different sections of the manuscript. JKP, LFF, MDPRT, RG, SS, SH and HSS edited the manuscript. All authors read and approved the final manuscript.
Acknowledgements
Jayanta Kumar Patra and Gitishree Das are grateful to Dongguk University, Republic of Korea for support. Leonardo Fernandes Fraceto and Estefânia V.R. Campos are grateful for the financial support provided by the São Paulo State Research Foundation (FAPESP) and National Council for Scientific and Technological Development (CNPQ). Maria del Pilar Rodriguez-Torres wishes to thank particularly DGAPA UNAM for the postdoctoral scholarship granted. Maria del Pilar Rodriguez-Torres, Laura Susana Acosta-Torres and Luis Armando Diaz-Torres thank Red de Farmoquimicos-CONACYT and DGAPA-UNAM PAPIIT-IN225516 project for support. Renato Grillo would like to thanks the São Paulo State Science Foundation (FAPESP, Grants #2015/26189-8). Han-Seung Shin thank Korea Environmental Industry & Technology Institute (A117-00197-0703-0) and Korea Institute of Planning and Evaluation for Technology in Food, Agriculture, Forestry and Fisheries (IPET) through Agricultural-BioTechnology Development Program funded by Ministry of Agriculture, Food and Rural Affairs (MAFRA)(710 003-07-7- SB120, 116075-3) for funding.
Competing interests
The authors declare that they have no competing interests.
Availability of data and materials
Not applicable.
Consent for publication
We have included two figures (Figs. 3 and 6 ) and one table (Table 1 ) from previously published literature with required copyright permission from the copyright holder. We have mentioned this in the manuscript with proper citation.
Ethics approval and consent to participate
This work was supported by the Korea Environmental Industry & Technology Institute (A117-00197-0703-0) and Korea Institute of Planning and Evaluation for Technology in Food, Agriculture, Forestry and Fisheries (IPET) through Agricultural-BioTechnology Development Program funded by Ministry of Agriculture, Food and Rural Affairs (MAFRA)(710 003-07-7- SB120, 116075-3).
Publisher’s Note
Springer Nature remains neutral with regard to jurisdictional claims in published maps and institutional affiliations.
Author information
Authors and affiliations.
Research Institute of Biotechnology & Medical Converged Science, Dongguk University-Seoul, Goyang-si, 10326, Republic of Korea
Jayanta Kumar Patra & Gitishree Das
Sao Paulo State University (UNESP), Institute of Science and Technology, Sorocaba, São Paulo, Zip Code 18087-180, Brazil
Leonardo Fernandes Fraceto & Estefania Vangelie Ramos Campos
Department of Biochemistry and Tissue Biology, Institute of Biology, State University of Campinas, Campinas, São Paulo, Zip code 13083-862, Brazil
Laboratorio de Investigación Interdisciplinaria, Área de Nanoestructuras y Biomateriales, Escuela Nacional de Estudios Superiores, Unidad Leon, Universidad Nacional Autonóma de México (UNAM), Boulevard UNAM No 2011. Predio El Saucillo y El Potrero, 37684, León, Guanajuato, Mexico
Maria del Pilar Rodriguez-Torres & Laura Susana Acosta-Torres
Centro de Investigaciones en Óptica, A.P. 1-948, C.P. 37000, León, Guanajuato, Mexico
Luis Armando Diaz-Torres
Department of Physics and Chemistry, School of Engineering, São Paulo State University (UNESP), Ilha Solteira, SP, 15385-000, Brazil
Renato Grillo
Department of Crop Science, Faculty of Agriculture, Universiti Putra Malaysia, 43400, Serdang, Selangor, Malaysia
Mallappa Kumara Swamy
Department of Biotechnology, Motilal Nehru National Institute of Technology Allahabad, Allahabad, Uttar Pradesh, 211004, India
Shivesh Sharma
Pharmacognosy Research Laboratories & Herbal Analysis Services UK, University of Greenwich, Medway Campus-Science, Grenville Building (G102/G107), Central Avenue, Chatham-Maritime, Kent, ME4 4TB, UK
Solomon Habtemariam
Department of Food Science and Biotechnology, Dongguk University, Ilsandong-gu, Goyang, Gyeonggi-do, 10326, Republic of Korea
Han-Seung Shin
You can also search for this author in PubMed Google Scholar
Corresponding author
Correspondence to Han-Seung Shin .
Rights and permissions
Open Access This article is distributed under the terms of the Creative Commons Attribution 4.0 International License ( http://creativecommons.org/licenses/by/4.0/ ), which permits unrestricted use, distribution, and reproduction in any medium, provided you give appropriate credit to the original author(s) and the source, provide a link to the Creative Commons license, and indicate if changes were made. The Creative Commons Public Domain Dedication waiver ( http://creativecommons.org/publicdomain/zero/1.0/ ) applies to the data made available in this article, unless otherwise stated.
Reprints and permissions
About this article
Cite this article.
Patra, J.K., Das, G., Fraceto, L.F. et al. Nano based drug delivery systems: recent developments and future prospects. J Nanobiotechnol 16 , 71 (2018). https://doi.org/10.1186/s12951-018-0392-8
Download citation
Received : 19 July 2018
Accepted : 25 August 2018
Published : 19 September 2018
DOI : https://doi.org/10.1186/s12951-018-0392-8
Share this article
Anyone you share the following link with will be able to read this content:
Sorry, a shareable link is not currently available for this article.
Provided by the Springer Nature SharedIt content-sharing initiative
- Nanomedicine
- Nanomaterials
- Drug delivery
- Drug targeting
- Natural products
Journal of Nanobiotechnology
ISSN: 1477-3155
- Submission enquiries: [email protected]
- Research article
- Open access
- Published: 15 April 2020
A drug identification model developed using deep learning technologies: experience of a medical center in Taiwan
- Hsien-Wei Ting 1 , 2 ,
- Sheng-Luen Chung 3 ,
- Chih-Fang Chen 4 ,
- Hsin-Yi Chiu 3 &
- Yow-Wen Hsieh 5
BMC Health Services Research volume 20 , Article number: 312 ( 2020 ) Cite this article
15k Accesses
25 Citations
Metrics details
Issuing of correct prescriptions is a foundation of patient safety. Medication errors represent one of the most important problems in health care, with ‘look-alike and sound-alike’ (LASA) being the lead error. Existing solutions to prevent LASA still have their limitations. Deep learning techniques have revolutionized identification classifiers in many fields. In search of better image-based solutions for blister package identification problem, this study using a baseline deep learning drug identification (DLDI) aims to understand how identification confusion of look-alike images by human occurs through the cognitive counterpart of deep learning solutions and thereof to suggest further solutions to approach them.
We collected images of 250 types of blister-packaged drug from the Out-Patient Department (OPD) of a medical center for identification. The deep learning framework of You Only Look Once (YOLO) was adopted for implementation of the proposed deep learning. The commonly-used F1 score, defined by precision and recall for large numbers of identification tests, was used as the performance criterion. This study trained and compared the proposed models based on images of either the front-side or back-side of blister-packaged drugs.
Our results showed that the total training time for the front-side model and back-side model was 5 h 34 min and 7 h 42 min, respectively. The F1 score of the back-side model (95.99%) was better than that of the front-side model (93.72%).
Conclusions
In conclusion, this study constructed a deep learning-based model for blister-packaged drug identification, with an accuracy greater than 90%. This model outperformed identification using conventional computer vision solutions, and could assist pharmacists in identifying drugs while preventing medication errors caused by look-alike blister packages. By integration into existing prescription systems in hospitals, the results of this study indicated that using this model, drugs dispensed could be verified in order to achieve automated prescription and dispensing.
Peer Review reports
Issuing of correct prescriptions is the mainstay of patient safety. Medication errors are the most important problem that influences safety in health care [ 1 ]. The most common medication errors are caused by human factors, such as fatigue and inadequate knowledge [ 2 ]. In particular, look-alike and sound-alike (LASA) is the lead error at the level of pharmacists or physicians. A good policy to prevent LASA is to change drug names and their packaging [ 3 ]. Researchers used chart reviews and mathematical methods to identify problematic pairs of drug names, and constructed an automated detection system to detect and prevent LASA errors [ 4 ]. Unfortunately, major problems remain in drug identification: many drugs look alike; drugs are relatively small in size; and a large number of drugs need to be identified. Existing identification solutions still have their limitations [ 5 , 6 , 7 ].
However, some assistive tools do exist. Automated dispensing cabinets (ADCs) represent a solution that dispenses drugs automatically [ 8 , 9 ], and there are many ADC technologies in existence. Some studies have used barcoding for drug identification and prevention of medication errors [ 9 ]. Devices that employ radio-frequency identification (RFID) and Bluetooth to identify the positions of drugs have been designed [ 8 ]. Most large hospitals use robots; however, there are fewer robots than needed in hospitals with fewer than 100 beds [ 10 ]. Other major problems with the use of ADCs are the development of suitable software that can identify drugs accurately without the need for pre-processing of drugs or a large space in the pharmaceutical department before applying the systems. In addition, it needs to be ensured that these systems will not increase the burden on pharmacists during the prescription process [ 11 , 12 ].
Alternatively, image-based solutions have been developed. Traditional image recognition finds features through algorithms and then classifies images using certain classifiers [ 13 , 14 ]. Lee et al. encoded color and shape into a three-dimensional histogram and geometric matrix, and encoded the imprint as a feature vector through a Scale Invariant Feature Transform (SIFT) descriptor and a Multi-scale Local Binary Pattern (MLBP) [ 15 ]. Taran et al. [ 16 ] proposed the use of a variety of traditional artificial feature integration methods to extract high-dimensional drug features from images to achieve identification of blister packages. Saitoh [ 17 ] used the local feature and nearest-neighbor search method to sort images of blister packages in a database according to input test images, and sorted blister packages with the most similar shapes and colors through voting scores.
Most significantly, thanks to the vigorous development of Graphics Processing Units (GPUs) for parallel computing, a current mainstream process is to adopt deep learning methods to replace traditional classifiers. Examples include biomedical imaging and wave recognition [ 18 , 19 ]; speech recognition [ 20 , 21 ]; biomedical signal detection [ 18 , 19 , 22 ]; cancer identification [ 19 , 22 , 23 ]; potential drug discovery [ 24 , 25 ]; and adverse drug effects [ 26 ]. Images of the drug are pre-processed to obtain the correct viewing angle and drug separation, and the characteristics of the pills are established manually [ 27 ]. Drug identification is implemented in a framework based on a Deep Convolutional Network (DCN), and achieved good recognition. In addition, another method of pill identification first finds the location and area of the drug by detecting the edge contour of the pill [ 27 ]; then, through a variety of data augmentation methods such as color shift, size adjustment, Gaussian blur, etc., more training samples are generated to solve the problem of sparse training samples. Three GoogLeNet deep learning networks are used as the main classifiers to train the color, shape and characteristics of the pills, and the recognition results of the three models are then combined to obtain the final recognition results [ 28 ].
This study focused on the problem of drug identification using visual images of blister packages. We constructed a Deep Learning Drug Identification (DLDI) model that identifies drugs automatically and can assist pharmacists in dispensing prescriptions correctly. Our goal was to illustrate how ‘look-alike’ error can be captured and explained by a convolution-based deep learning network whose working mechanism is in much similarity to the human visionary recognition capability. Subsequently, appropriate solution to extract more detailed nuance differences can be utilized in distinguishing look-alike objects.
To investigate how a deep learning network identifies object types, a dataset containing images both sides of 250 types of blister packages were collected for training and testing data of a deep learning network. Identification results in terms of precision, recall, and the combined F1-score were computed, where an identification error can be regarded as an error due to look-alike cases.
Data resources
This study collected drugs from the Out-Patient Department (OPD) of a medical center. Of the 272 kinds of drug, this study focused only on recognition of pharmaceutical blister packages. As such, 6 classes of drug packaging (Fig. 1 ), totaling 32 kinds of drug, were excluded, as follows: clip chain bags, powder bags, foil packaging bags, transparent bags, paper packages, and bottle packaging. The remaining 250 drugs with blister packaging were considered.
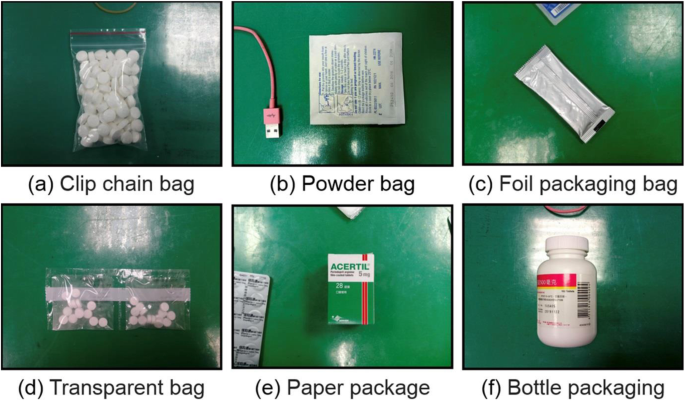
Excluded drug packages. Six classes of drug packaging were excluded: clip chain bags, powder bags, foil packaging bags, transparent bags, paper packages, and bottle packaging
We aimed to identify blister packages by their images, photographed using a camera from different angles. In collecting the training set, 72 images were taken for each side of each type of drug: the camera focused from 9 different angles, with 8 different rotation directions of the drug shown in the images (Fig. 2 ). Both front-side and back-side images were taken for each drug, resulting in a total of 36,000 images as the training data for deep learning. Images of the front sides of packages contained the shapes and colors of the pills or tablets, whereas images of the back sides contained mostly texture patterns of the drugs or logos of pharmaceutical companies. These images were used to train CNN networks, the deep learning networks, for object identification.
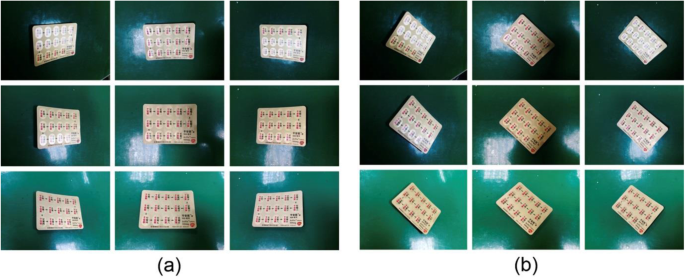
Photographs from different angles . Different angles were employed for the camera to focus on, with different rotation directions of the drug packaging. Both front-side and back-side images were obtained for each type of drug
Deep learning architecture
The concept of the Convolution Neural Network (CNN) was proposed by LeCun and others in 1989. These deep learning networks usually consist of convolutional layers, pooling layers and fully-connected layers [ 29 ]. As the convolutional layers and the pooling layers in the network architecture enhance the relationship between pattern recognition and adjacent data, a CNN can be applied to signal types such as images and sounds. Through multi-layer convolution and pooling, the extracted features are treated as inputs, and then forwarded to one or more fully-connected layers for classification. Unfortunately, the simple CNN is not effective for more complex images. Krizhevsky et al. [ 30 ] reconstructed a CNN in 2012, and in CNN-based networks, the deep learning framework of “object detection” has also been continuously improved. R-CNN was the first successful CNN-based object detection method, but the speed of detection was very slow [ 31 ]. Later, the Fast and Faster R-CNN were constructed [ 32 ], optimized on the basis of R-CNN, and the speed and accuracy were improved significantly.
Software and hardware devices
This study used You Only Look Once (the abbreviation ‘YOLO’ having been proposed by Redmon et al. in 2015) as the solution framework for deep learning [ 33 ]. An end-to-end structure was adopted, and compared with the general deep learning method, YOLO focuses on both the area prediction part of detection and the category prediction part for classification. YOLO integrates detection and classification into the same neural network model, with fast and accurate target detection and recognition. These deep learning techniques employ the following features: batch normalization for faster convergence; passthrough for the features identification increasing; hi-res classifier to increase the resolution of the images; direct location prediction to strengthen the stabilization of position prediction; and multi-scale training to improve both speed and accuracy. The SENet and ResNet experiments in this study used the Kubuntu 14.04 system and the Darknet framework in the Caffe structure of Windows 7, which is a special hardware device host for deep learning. This study also employed an Intel® I7–6770 Eight-Core Processor (CPU), 16 GB RAM, and a NVIDIA GTX 1080 Graphic Processing Unit (GPU).
Experimental design
For model evaluation, this study partitioned the collected data into separate training and testing sets. The training set trained the deep network to generate models, while the testing set evaluated the performance of the constructed models. We randomly choose three-quarters of the 72 pictures of each type of drug for inclusion in the training set, and the remaining quarter were included in the testing set, with 13,500 images in total in the training set and 4500 images in the testing set. This study trained 100 models for each of the front-side and back-side images using the training set. The best model was chosen, which was defined as the model with the greatest accuracy (highest F1 measure) and the fastest speed (fewest Epochs). This study also standardized the YOLO v2 protocol for both the training and testing datasets in each model. All images were converted into 224 × 224 pixels. Neither data augmentation nor pre-training of the model were performed during training. The batch size was 8, meaning that parameters were re-adjusted every 8 images. The highest training frequency was 100 Epochs, one Epoch meaning that the deep network ran all the pictures during training. The parameters were saved after every Epoch was completed (Table 1 ).
Outcome measurement
Confusion matrixes were used to record the results if blister packages were identified, correctly or not. Correct matches were listed on the diagonal of the matrix, whereas cases of missed identification were marked by non-zero values off the diagonal. The higher the number, the greater the chance of misidentification of blister packages of drugs. For example, assume that there is a system for classifying three different drugs (Table 3 ). Suppose that there are 28 drugs in total: 9 drug A, 6 drug B, and 13 drug C. In this confusion matrix, there are actually nine drug A, but three of them are misidentified as drug B. For drug B, one of the drugs is misidentified as drug C, and two are misidentified as drug A. The confusion matrix shows that it is more difficult to distinguish between drug A and drug B, but easier to distinguish drug C from the other drugs.
The data presented in Table 2 are for the model obtained from 100-Epoch training. The training time, number of training Epochs, precision, recall, and F1 measure were recorded as the evaluation results. The best recognition performance was identified according to the F1 score, and the Epoch number was used to identify the fewest numbers of training Epochs. The recall, also called the true positive rate or the sensitivity, measures the proportion of positives correctly identified. Recall = True Positive / (True Positive + False Negative), of which True Positive denotes a correct identification; while False Negative denotes a misidentified result by taking the correct target as something else. The precision, also called the positive predictive value, measures the proportion of positives among all identified. Precision = True Positive / (True Positive + False Positive), of which False Positive denote a misidentified result by taking something else as the correct target [ 12 ]. The F1 measure is an evaluation that combines both sensitivity (recall) and precision. The calculation formula of the F1 score is as follows:
At the same time, we recorded the training time of the model, the number of Epochs in the training, and the classification performance of the model for the testing dataset.
In this study, two deep learning models were employed for training, and the identification results were compared: the front-side (pill shape and color) model and the back-side (textual pattern and logo) model of blister-packaged drugs. The total training time of the front-side model and the back-side model was 5 h 34 min and 7 h 42 min, respectively. The number of Epochs of the front-side model and the back-side model was 60 and 65, respectively. The precision and recall of the back-side model (96.26 and 96.63%, respectively) were better than those of the front-side model (94.09 and 94.44%, respectively), meaning that texture and logo carried more distinguishing features than were contained in pill shape and color. The F1 score of the back-side model (95.99%) was better than that of the front-side model (93.72%) (Table 2 ), meaning that when only one model can be used, the back-side model is the preferred choice.
In order to show that the identification performance of the deep learning network for blister-package identification is comparable to that of the human eye, we used the YOLO v2 testing line chart to illustrate the results for the front-side and back-side images (Fig. 3 ). We found that the F1 score increased and the correct rate of identification increased as the training Epoch number increased; a plateau was then reached when the Epoch number was larger than 8–10, irrespective of front-side or back-side model.
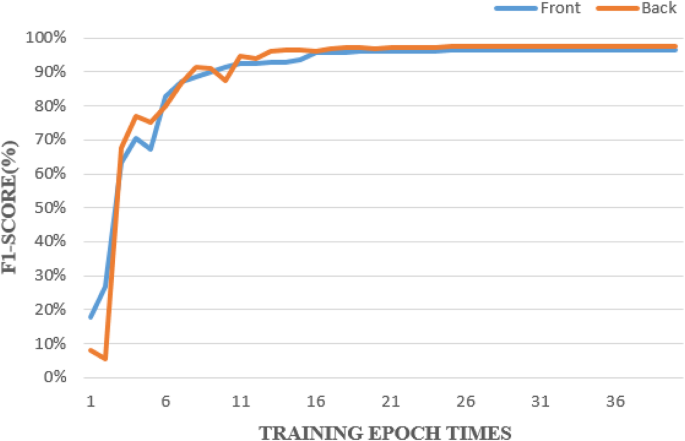
YOLO v2 testing line chart. The F1 score and the correctness rate of identification increased as the number of training Epochs increased; a plateau was then reached when the Epoch number was larger than 8–10, irrespective of front-side or back-side model
Deep learning models share cognitive capabilities similar to those of the human eye, and what confuses a deep learning network can also confuse the human eye. As such, in order to identify look-alike blister packages, we created confusion matrixes, which recorded the actual blister packages that were identified, correctly or not. Correct matches were listed on the diagonal of the matrix, whereas cases of missed identification were marked by non-zero values off the diagonal. The higher the number, the greater the chance of misidentification of blister packages of drugs.
For example, assume that there is a system for classifying three different drugs (Table 3 ). Suppose that there are 28 drugs in total: 9 drug A, 6 drug B, and 13 drug C. In this confusion matrix, there are actually nine drug A, but three of them are misidentified as drug B. For drug B, one of the drugs is misidentified as drug C, and two are misidentified as drug A. The confusion matrix shows that it is more difficult to distinguish between drug A and drug B, but easier to distinguish drug C from the other drugs. In the confusion matrix, correct identifications are on the diagonal; in contrast, misidentified ones are the non-zero terms off the diagonal.
This study identified two groups of misidentified images based on the confusion matrixes of the two experiments for the front-side and back-side models. According to the identification results recorded in the confusion matrix for the front-side model, the drug RITALIN (METHYLPHENIDATE) (Fig. 4 a) has a blister package that was misidentified as amBROXOL (MUSCO) (Fig. 4 b). In addition, the blister package of ATENOLOL (UROSIN) (Fig. 4 c) was misidentified as DIHYDROEROGOTOXINE (Fig. 4 d). This is because the pills shown on the front of the blister packages share the same color and shape, leading to misidentification.
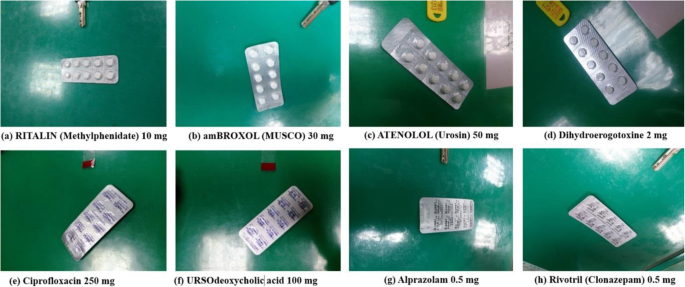
Front and back images of blister packages that were misidentified using the models. The identification results were recorded in confusion matrixes for each model. RITALIN (METHYLPHENIDATE) ( a ) was misidentified as amBROXOL (MUSCO) ( b ) and ATENOLOL (UROSIN) ( c ) was misidentified as DIHYDROEROGOTOXINE ( d ) in the front-side model, while Ciprofloxacin ( e ) was misidentified as URSOdeoxycholic acid (Fig. 4f) and Alprazolam ( g ) was misidentified as Rivotril (CLONAZEPAM) ( h ) in the back-side model.
According to the identification results recorded in the confusion matrix for the back-side model, the blister package of Ciprofloxacin (Fig. 4 e) was misidentified as URSOdeoxycholic acid (Fig. 4 f), and the blister package of Alprazolam (Fig. 4 g) was misidentified as Rivotril (CLONAZEPAM) (Fig. 4 h). These two misidentifications were due to the fact that the backs of the blister packages were wrapped in aluminum foil, and the textual patterns on the back-side were of the same color, without significant difference.
The study provides a qualitative examination regarding how look-alike blister packages are recognized or constrained by deep learning networks that are reminiscent of human visionary cognition capability. With racing speed of progress in deep learning techniques, it is expected that more accurate deep learning solutions will emerge to distinguish nuance image features among different object types, thus mitigating if not solving the dispensing error caused by look-alike blister packages.
Image based techniques, being non-intrusive and without resort to additional devices like RFID tag or bar code, have been a preferred solution to object identification problems. Traditional image-based solutions by computer vision rely on well-defined hierarchical features for effective comparison [ 34 , 35 ]. Some of the research work from literature reported performance of less than 80% of accuracy with limited number of types of less than 50 [ 15 , 36 ]. In contrast, the distinguishing features reported in this study are learned by adjusting network parameters through fitting training data, the process being much similar to human visionary recognition process, thus achieving accuracy better than 90% among 250 types. With the advent of deep learning technique, identification witnesses a revolutionary shift which can benefit blister package identification critical to dispensing safety.
This study proposed a novel deep learning drug identification (DLDI) model that delivered satisfactory results for drug identification based on images of blister packages. The results of this study showed that identification by “deep learning” is no less accurate than identification by the human eye. The CNN simulates the response of neurons in the human brain to signals by performing various mathematical operations on features to complete the classification. Repetition of these processes achieves the purpose of recognition. In earlier studies, features were defined subjectively to identify blister packages of drugs [ 17 ]. Deep learning allows learning of features automatically, without the need to define features of drugs before machine learning. This advantage eliminates human error and assists pharmacists to identify drugs correctly. Deep learning enables identification of the characteristics of individual drugs clearly and recognizes the drugs that pharmacists/humans consider look alike. Just one or two cameras in dispensing cabinets are required, and medication errors will be prevented.
Referring to Table 2 , this study found that back-side images of blister packages of drugs were better than front-side images for identification purposes. While back-side took more training time to better distinguish textual features, based on 4500 test images evenly distributed over 250 types, the associated performance criteria of: precision, recall, and F1 score are all better than that by the front-side images. This is because the information on the back of the packages includes the pharmaceutical company, drug name, dose, and logo in larger text than on the front of the package, which only presents information regarding the color and shape of the pills. The front of the drug packaging contains some three-dimensional information with regards to drug shape. However, some blister packages were not easily recognized by the deep learning network, and were more likely to be confused according to the confusion matrix. These unrecognizable blister packages correspond to look-alike blister packages recognized by the human eye. In the future, we will employ a convolution kernel to identify data features to generate signals and perform a comparison with the human eye.
There are many kinds of drug packages that need to be identified: pills; blister packaging; clip chain bags; powder bags; foil packaging bags; transparent bags; paper packages; bottle packaging, etc. For medication adherence and drug preservation, most drugs are packaged in blisters [ 37 ]. Moreover, for some drugs, infrared spectrum analysis of tablets in intact blisters is performed to distinguish between genuine and counterfeit samples [ 38 ]. DLDI models may also be applied to automated dispensing cabinets (ADCs), and can be employed in cooperation with both pharmacists and robots. Some robots have cameras, which would be useful for application of our model for drug identification. In the future, we will construct a blister-package identification model that takes account of both sides of the packaging, which will contain more information than just a single side for identification. The identification accuracy may also be increased by use of three-dimensional images of drugs or images with different spectrums for deep learning.
There are some considerations for future studies. First, this study only examined blister-packaged drugs, and used the whole of the blister packages for identification. This model cannot be used to identify blister packages when held in the hand, or trimmed blister packages. Moreover, other types of drug packaging need to be studied. In some cases, the pill size and shape were too familiar to identify. One of the aims of future study is to address these issues. Second, the training time was too long, with more than 5 h required for training the models in this study. More time is required if more than one kind of spectrum is used, and a more effective program is needed to train the models. Third, re-training would be needed if one or more new drugs are added in this model. In the future, we hope to develop a system in which only “PARTIAL” training is required when drugs are changed or added.
Our goal was to illustrate how ‘look-alike’ error can be captured and explained by a convolution-based deep learning network whose working mechanism is in much similarity to the human visionary recognition capability. Subsequently, appropriate solution to extract more detailed nuance differences can be utilized in distinguishing look-alike objects. With an accuracy greater than 90%, the results of this study may be applied to the real environment, and may assist pharmacists to identify drugs and prevent medication errors caused by look-alike blister packages. The results of this study can also form the core software for robots, allowing filling of prescriptions automatically and preventing medication errors.
Availability of data and materials
The datasets generated and analyzed during the current study are not publicly available due to that was belonged to the MacKay Memorial Hospital but are available from the corresponding author on reasonable request.
Abbreviations
Automated dispensing cabinets
Convolution Neural Network
Deep Convolutional Network
Deep learning drug identification
Graphics Processing Units
Look-alike and sound-alike
Multi-scale Local Binary Pattern
Out-Patient Department
Radio-frequency identification
Scale Invariant Feature Transform
You Only Look Once
Gates PJ, Baysari MT, Mumford V, Raban MZ, Westbrook JI. Standardising the classification of harm associated with medication errors: the harm associated with medication error classification (HAMEC). Drug Saf. 2019;42(8):931–9.
Article Google Scholar
Mekonnen AB, Alhawassi TM, McLachlan AJ, Brien JE. Adverse drug events and medication errors in African hospitals: a systematic review. Drugs Real World Outcomes. 2018;5(1):1–24.
Zhong W, Feinstein JA, Patel NS, Dai D, Feudtner C. Tall man lettering and potential prescription errors: a time series analysis of 42 children's hospitals in the USA over 9 years. BMJ Qual Saf. 2016;25(4):233–40.
Rash-Foanio C, Galanter W, Bryson M, Falck S, Liu KL, Schiff GD, Vaida A, Lambert BL. Automated detection of look-alike/sound-alike medication errors. Am J Health Syst Pharm. 2017;74(7):521–7.
Tseng HY, Wen CF, Lee YL, Jeng KC, Chen PL. Dispensing errors from look-alike drug trade names. Eur J Hosp Pharm. 2018;25(2):96–9.
Kang HJ, Park H, Oh JM, Lee EK. Perception of reporting medication errors including near-misses among Korean hospital pharmacists. Medicine. 2017;96(39):e7795.
Aldhwaihi K, Schifano F, Pezzolesi C, Umaru N. A systematic review of the nature of dispensing errors in hospital pharmacies. Integr Pharm Res Pract. 2016;5:1–10.
Testa M, Pollard J. Safe pill-dispensing. Stud Health Technol Inform. 2007;127:139–46.
PubMed Google Scholar
Harolds JA, Harolds LB. Quality and safety in health care, part X: other technology to reduce medication errors. Clin Nucl Med. 2016;41(5):376–8.
Pedersen CA, Schneider PJ, Scheckelhoff DJ. ASHP national survey of pharmacy practice in hospital settings: dispensing and administration--2014. Am J Health Syst Pharm. 2015;72(13):1119–37.
Johnson CL, Carlson RA, Tucker CL, Willette C. Using BCMA software to improve patient safety in veterans administration medical centers. J Healthc Inf Manag. 2002;16(1):46–51.
Wang J-S, Ambikapathi A, Han Y, Chung S-L, Ting H-W, Chen C-F. Highlighted Deep Learning based Identification of Pharmaceutical Blister Package. In: IEEE 23rd International Conference on Emerging Technologies and Factory Automation (ETFA 2018) ; 2018.
Google Scholar
Suntronsuk S, Ratanotayanon S. Automatic text imprint analysis from pill images. In: 2017 9th International Conference on Knowledge and Smart Technology: Crunching Information of Everything, KST ; 2017. p. 288–93.
Chapter Google Scholar
Chen R-C, Chan Y-K, Chen Y-H, Bau C-T. An automatic drug image identification system based on multiple image features and dynamic weights. Int J Innovative Comput Inf Control. 2012;8(5):2995–3013.
Lee YB, Park U, Jain AK, Lee SW. Pill-ID: matching and retrieval of drug pill images. Pattern Recogn Lett. 2012;33(7):904–10.
Taran O, Rezaeifar S, Dabrowski O, Schlechten J, Holotyak T, Voloshynovskiy S. PharmaPack: mobile fine-grained recognition of pharma packages. Eur Signal Pr Conf. 2017;1:1917–21.
Saitoh K. Pharmaceutical Blister Pack Recognition using Local Features. In: MVA2013 IAPR International Conference on Machine Vision Applications . Kyoto; 2013. p. 355–8.
Li Y, Huang C, Ding L, Li Z, Pan Y, Gao X. Deep learning in bioinformatics: introduction, application, and perspective in the big data era. Methods. 2019;166:4–21.
Article CAS Google Scholar
Ravi D, Wong C, Deligianni F, Berthelot M, Andreu-Perez J, Lo B, Yang GZ. Deep learning for health informatics. IEEE J Biomed Health Inform. 2017;21(1):4–21.
Sainath TN, Kingsbury B, Saon G, Soltau H, Mohamed AR, Dahl G, Ramabhadran B. Deep convolutional neural networks for large-scale speech tasks. Neural Netw. 2015;64:39–48.
Lopez-de-Ipina K, Martinez-de-Lizarduy U, Calvo PM, Mekyska J, Beitia B, Barroso N, Estanga A, Tainta M, Ecay-Torres M. Advances on automatic speech analysis for early detection of Alzheimer disease: a non-linear multi-task approach. Curr Alzheimer Res. 2018;15(2):139–48.
Zou L, Yu S, Meng T, Zhang Z, Liang X, Xie Y. A technical review of convolutional neural network-based mammographic breast Cancer diagnosis. Comput Math Methods Med. 2019;2019:6509357.
Deng W, Shi Q, Luo K, Yang Y, Ning N. Brain tumor segmentation based on improved convolutional neural network in combination with non-quantifiable local texture feature. J Med Syst. 2019;43(6):152.
Ekins S, Puhl AC, Zorn KM, Lane TR, Russo DP, Klein JJ, Hickey AJ, Clark AM. Exploiting machine learning for end-to-end drug discovery and development. Nat Mater. 2019;18(5):435–41.
You J, McLeod RD, Hu P. Predicting drug-target interaction network using deep learning model. Comput Biol Chem. 2019;80:90–101.
Gao M, Igata H, Takeuchi A, Sato K, Ikegaya Y. Machine learning-based prediction of adverse drug effects: an example of seizure-inducing compounds. J Pharmacol Sci. 2017;133(2):70–8.
Wong YF, Ng HT, Leung KY, Chan KY, Chan SY, Loy CC. Development of fine-grained pill identification algorithm using deep convolutional network. J Biomed Inform. 2017;74:130–6.
Szegedy C, Liu W, Jia YQ, Sermanet P, Reed S, Anguelov D, Erhan D, Vanhoucke V, Rabinovich A. Going deeper with convolutions. Proc Cvpr Ieee. 2015;1:1–9.
Lecun Y, Bottou L, Bengio Y, Haffner P. Gradient-based learning applied to document recognition. P Ieee. 1998;86(11):2278–324.
Krizhevsky A, Sutskever I, Hinton GE. ImageNet classification with deep convolutional neural networks. Commun ACM. 2017;60(6):84–90.
Girshick R, Donahue J, Darrell T, Malik J. Rich feature hierarchies for accurate object detection and semantic segmentation. IEEE Conf Comput Vision Pattern Recognit (Cvpr). 2014;2014:580–7.
Ren SQ, He KM, Girshick R, Sun J. Faster R-CNN: towards real-time object detection with region proposal networks. IEEE Trans Pattern Anal Mach Intell. 2017;39:1137–49.
Redmon J, Divvala S, Girshick R, Farhadi A. You only look once: unified, real-time object detection. IEEE Conf Compute Vision Pattern Recognit (Cvpr). 2016;2016:779–88.
Wang Y, Ribera J, Liu C, Yarlagadda S, Zhu F. Pill Recognition Using Minimal Labeled Data. In: 2017 IEEE Third International Conference on Multimedia Big Data (BigMM) ; 2017. p. 346–53.
Girshick R, Donahue J, Darrell T, Malik J. Rich feature hierarchies for accurate object detection and semantic segmentation. Proc IEEE Conf Comput Vis Pattern Recognit. 2014;1:580.
Pardo CE, Sosa LF, Gutierrez EA, Jiménez FR. Classification system for blister pack of pills. In: 2014 IEEE 5th Colombian Workshop on Circuits and Systems (CWCAS) ; 2014. p. 1–6.
Gilmartin JFM, Hussainy SY, Marriott JL. Medicines in Australian nursing homes: a cross-sectional observational study of the accuracy and suitability of re-packing medicines into pharmacy-supplied dose administration aids. Res Soc Adm Pharm. 2013;9(6):876–83.
Custers D, Vandemoortele S, Bothy JL, De Beer JO, Courselle P, Apers S, Deconinck E. Physical profiling and IR spectroscopy: simple and effective methods to discriminate between genuine and counterfeit samples of Viagra (R) and Cialis (R). Drug Test Anal. 2016;8(3–4):378–87.
Download references
Acknowledgements
Not applicable.
This study was funded by Mackay Memorial Hospital and the National Taiwan University of Science and Technology Corporation Program, funding reference MMH-NTUST-106-05.
Author information
Authors and affiliations.
Department of Neurosurgery, Taipei Hospital, Ministry of Health and Welfare, New Taipei City, Taiwan
Hsien-Wei Ting
Graduate Program in Biomedical Informatics, Yuan Ze University, Taoyuan City, Taiwan
Department of Electrical Engineering, National Taiwan University of Science and Technology, Taipei City, Taiwan
Sheng-Luen Chung & Hsin-Yi Chiu
Pharmaceutical Department, Mackay Memorial Hospital, No. 92, Sec. 2, Zhongshan N. Rd, Taipei City, 10449, Taiwan
Chih-Fang Chen
Department of Pharmacy, China Medical University Hospital, Taichung, Taiwan
Yow-Wen Hsieh
You can also search for this author in PubMed Google Scholar
Contributions
HWT drafted the manuscript. HWT, SLC, CFC and YWH contributed to the study concept and design; SLC and HYC analyzed study data, and SLC and CFC interpreted study data. All authors gave final approval before submission.
Corresponding author
Correspondence to Chih-Fang Chen .
Ethics declarations
Ethics approval and consent to participate.
Permission to collect the data was obtained from Mackay Memorial Hospital.
Consent for publication
Not applicable: no individual person’s data.
Competing interests
The authors declare that they have no competing interest.
Additional information
Publisher’s note.
Springer Nature remains neutral with regard to jurisdictional claims in published maps and institutional affiliations.
Rights and permissions
Open Access This article is licensed under a Creative Commons Attribution 4.0 International License, which permits use, sharing, adaptation, distribution and reproduction in any medium or format, as long as you give appropriate credit to the original author(s) and the source, provide a link to the Creative Commons licence, and indicate if changes were made. The images or other third party material in this article are included in the article's Creative Commons licence, unless indicated otherwise in a credit line to the material. If material is not included in the article's Creative Commons licence and your intended use is not permitted by statutory regulation or exceeds the permitted use, you will need to obtain permission directly from the copyright holder. To view a copy of this licence, visit http://creativecommons.org/licenses/by/4.0/ . The Creative Commons Public Domain Dedication waiver ( http://creativecommons.org/publicdomain/zero/1.0/ ) applies to the data made available in this article, unless otherwise stated in a credit line to the data.
Reprints and permissions
About this article
Cite this article.
Ting, HW., Chung, SL., Chen, CF. et al. A drug identification model developed using deep learning technologies: experience of a medical center in Taiwan. BMC Health Serv Res 20 , 312 (2020). https://doi.org/10.1186/s12913-020-05166-w
Download citation
Received : 24 October 2019
Accepted : 27 March 2020
Published : 15 April 2020
DOI : https://doi.org/10.1186/s12913-020-05166-w
Share this article
Anyone you share the following link with will be able to read this content:
Sorry, a shareable link is not currently available for this article.
Provided by the Springer Nature SharedIt content-sharing initiative
- Deep learning
- Drug identification
- Look-alike and sound-alike (lasa)
- Medication error
- Patient safety
BMC Health Services Research
ISSN: 1472-6963
- General enquiries: [email protected]
Thank you for visiting nature.com. You are using a browser version with limited support for CSS. To obtain the best experience, we recommend you use a more up to date browser (or turn off compatibility mode in Internet Explorer). In the meantime, to ensure continued support, we are displaying the site without styles and JavaScript.
- View all journals
Drug discovery articles from across Nature Portfolio
Drug discovery is the process through which potential new medicines are identified. It involves a wide range of scientific disciplines, including biology, chemistry and pharmacology.
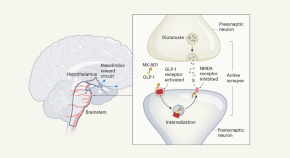
Dual-action obesity drug rewires brain circuits for appetite
A two-in-one drug that modulates neural pathways involved in appetite and reward might prove to be more effective and longer lasting than current weight-loss drugs on the market.
- Tyler M. Cook
- Darleen Sandoval
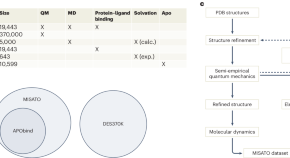
A multidimensional dataset for structure-based machine learning
MISATO, a dataset for structure-based drug discovery combines quantum mechanics property data and molecular dynamics simulations on ~20,000 protein–ligand structures, substantially extends the amount of data available to the community and holds potential for advancing work in drug discovery.
- Matthew Holcomb
- Stefano Forli
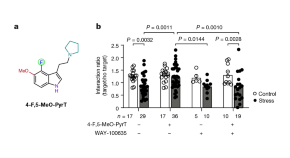
Toad psychedelic points to biological target for antidepressants
A hallucinogenic compound secreted by toads has served as a springboard for research into the therapeutic benefits of psychedelics. The findings suggest that these compounds exert antidepressant effects in part by binding an under-appreciated target in the brain.
Related Subjects
- Business strategy in drug development
- Diagnostics
- Drug delivery
- Drug regulation
- Drug safety
- Drug screening
- Medicinal chemistry
- Pharmaceutics
- Pharmacology
- Target identification
- Target validation
Latest Research and Reviews
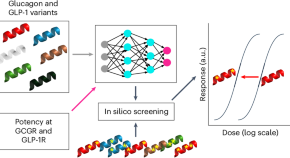
Machine learning designs new GCGR/GLP-1R dual agonists with enhanced biological potency
Engineering new ligands that specifically target multiple G protein-coupled receptors with desired activity profiles requires time-consuming and expensive cycles of design-make-test-analyse work. Now it has been shown that limited experimental data can be used to train sophisticated machine learning models to accurately predict the activity of previously uncharacterized peptide ligand variants.
- Anna M. Puszkarska
- Bruck Taddese
- Lucy J. Colwell
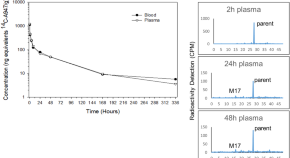
Tissue distribution and retention drives efficacy of rapidly clearing VHL-based PROTACs
Zhang et al. demonstrate that plasma sampling for a VHL-PROTAC does not represent the tissue concentrations necessary for efficacy; the tissue uptake/retention can be used to guide their therapeutic potential in target organs.
- Donglu Zhang
- Cornelis E. C. A. Hop
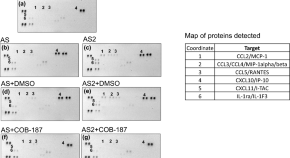
Mechanistic insights into SARS-CoV-2 spike protein induction of the chemokine CXCL10
- Davoud Ghazanfari
- Maria Cecilia Courreges
- Douglas J. Goetz
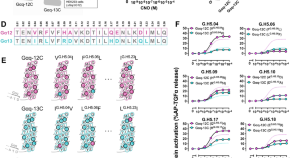
Identification of Gα 12 -vs-Gα 13 -coupling determinants and development of a Gα 12/13 -coupled designer GPCR
- Manae Tatsumi
- Christian Cruz
- Asuka Inoue
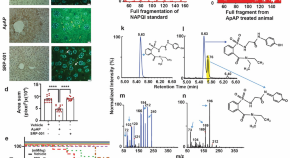
Transcriptomic signature, bioactivity and safety of a non-hepatotoxic analgesic generating AM404 in the midbrain PAG region
- Hernan A. Bazan
- Surjyadipta Bhattacharjee
- Nicolas G. Bazan
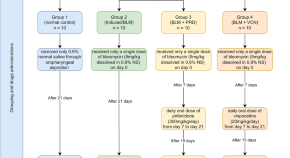
Vinpocetine alleviated alveolar epithelial cells injury in experimental pulmonary fibrosis by targeting PPAR-γ/NLRP3/NF-κB and TGF-β1/Smad2/3 pathways
- Zeena A. Hussein
- Ahmed R. Abu-Raghif
- Hayder Adnan Fawzi
News and Comment
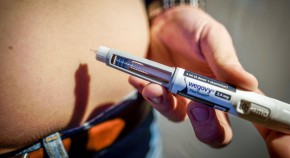
Experimental obesity drug packs double punch to reduce weight
Test of weight-loss candidate in mice shows that there is still room for improvement in a burgeoning field.
- Asher Mullard
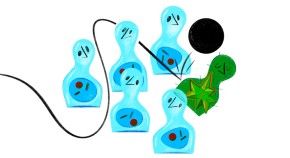
How to kill the ‘zombie’ cells that make you age
Researchers are using new molecules, engineered immune cells and gene therapy to kill senescent cells and treat age-related diseases.
- Carissa Wong
Advances in the management of achondroplasia
The first precision therapy for children with achondroplasia, vosoritide, is now approved in many regions, including Australia, the USA, the European Union and Japan. This article discusses the recent trial results for this therapy regarding growth and the co-morbidities associated with achondroplasia and considers the rationale for its clinical use.
- Ravi Savarirayan
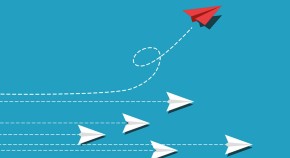
New route to target RAS
- M. Teresa Villanueva
Quick links
- Explore articles by subject
- Guide to authors
- Editorial policies

Breaking News
Star USC scientist faces scrutiny — retracted papers and a paused drug trial
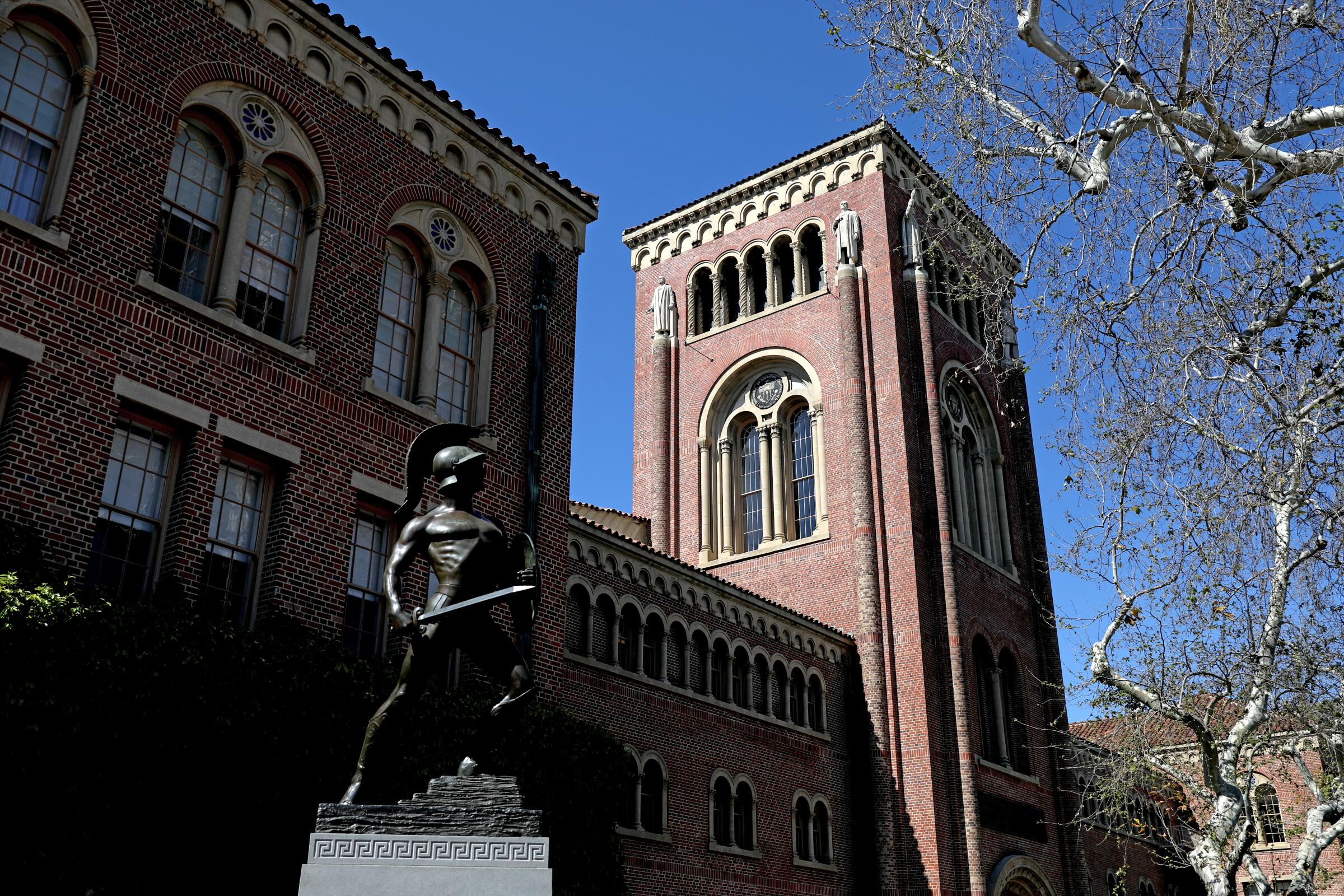
- Show more sharing options
- Copy Link URL Copied!
Late last year, a group of whistleblowers submitted a report to the National Institutes of Health that questioned the integrity of a celebrated USC neuroscientist’s research and the safety of an experimental stroke treatment his company was developing.
NIH has since paused clinical trials for 3K3A-APC, a stroke drug sponsored by ZZ Biotech, a Houston-based company co-founded by Berislav V. Zlokovic , professor and chair of the department of physiology and neuroscience at the Keck School of Medicine of USC.
Three of Zlokovic’s research papers have been retracted by the journal that published them because of problems with their data or images. Journals have issued corrections for seven more papers in which Zlokovic is the only common author, with one receiving a second correction after the new supplied data were found to have problems as well.
For an 11th paper co-authored by Zlokovic the journal Nature Medicine issued an expression of concern , a note journals append to articles when they have reason to believe there may be a problem with the paper but have not conclusively proven so. Since Zlokovic and his co-authors no longer had the original data for one of the questioned figures, the editors wrote, “[r]eaders are therefore alerted to interpret these results with caution.”
“Its quite unusual to see this volume of retractions, corrections and expressions of concern, especially in high-tier influential papers,” said Dr. Matthew Schrag, an assistant professor of neurology at Vanderbilt who co-authored the whistleblower report independently of his work at the university.
Both Zlokovic and representatives for USC declined to comment, citing an ongoing review initiated in the wake of the allegations, which were first reported in the journal Science.
“USC takes any allegations of research integrity very seriously,” the university said in a statement. “Consistent with federal regulations and USC policies, this review must be kept confidential.”
Science & Medicine
USC neuroscientist faces scrutiny following allegations of data manipulation
Accusations against USC’s Berislav Zlokovic were made by a small group of independent researchers and reported in the journal Science.
Nov. 24, 2023
Zlokovic “remains committed to cooperating with and respecting that process, although it is unfortunately required due to allegations that are based on incorrect information and faulty premises,” his attorney Alfredo X. Jarrin wrote in an email.
Regarding the articles, “corrections and retractions are a normal and necessary part of the scientific post-publication process,” Jarrin wrote.
Authors of the whistleblower report and academic integrity experts challenged that assertion.
“If these are honest errors, then the authors should be able to show the actual original data,” said Elisabeth Bik , a microbiologist and scientific integrity consultant who co-wrote the whistleblower report. “It is totally human to make errors, but there are a lot of errors found in these papers. And some of the findings are suggestive of image manipulation.”
Given the staid pace of academic publishing, publishing this many corrections and retractions only a few months after the initial concerns were raised “is, bizarrely, pretty quick,” said Ivan Oransky, co-founder of Retraction Watch .
The whistleblower report submitted to NIH identified allegedly doctored images and data in 35 research papers in which Zlokovic was the sole common author.
“There had been rumblings about things not being reproducible [in Zlokovic’s research] for quite some time,” Schrag said. “The real motivation to speak publicly is that some of his work reached a stage where it was being used to justify clinical trials. And I think that when you have data that may be unreliable as the foundation for that kind of an experiment, the stakes are just so much higher. You’re talking about patients who are often at the most vulnerable medical moment of their life.”
World & Nation
Countries struggle to draft ‘pandemic treaty’ to avoid mistakes made during COVID
After the devastation of the COVID-19 pandemic, the World Health Organization and leaders worldwide vowed to do better next time but are still struggling to finalize a global plan.
May 11, 2024
Over the years, Zlokovic has created several biotech companies aimed at commercializing his scientific work. In 2007, he co-founded ZZ Biotech , which has been working to gain federal approval of 3K3A-APC.
The drug is intended to minimize the bleeding and subsequent brain damage that can occur after an ischemic stroke, in which a blood clot forms in an artery leading to the brain.
In 2022, USC’s Keck School of Medicine received from NIH the first $4 million of a planned $30-million grant to conduct Phase III trials of the experimental stroke treatment on 1,400 people.
In Phase II of the trial, which was published in 2018 and called Rhapsody, six of the 66 patients who received 3K3A-APC died in the first week after their stroke, compared to one person among the 44 patients who got a placebo. Patients who received the drug also tended to report more disability 90 days after their stroke than those who got the placebo. The differences between the two groups were not statistically significant and could have been due to chance, and the death rate for patients in both groups evened out one month after the initial stroke.
“The statements that there is a risk in this trial is false,” said Patrick Lyden, a USC neurologist and stroke expert who was employed by Cedars-Sinai at the time of the trial. Zlokovic worked with Lyden as a co-investigator on the study.
One correction has been issued to the paper describing the Phase II results, fixing an extra line in a data table that shifted some numbers to the wrong columns. “This mistake is mine. It’s not anybody else’s. I didn’t catch it in multiple readings,” Lyden said, adding that he noticed the error and was already working on the correction when the journal contacted him about it.
He disputed that the trial represented any undue risk to patients.
“I believe it’s safe, especially when you consider that the purpose of Rhapsody was to find a dose — the maximum dose — that was tolerated by the patients without risk, and the Rhapsody trial succeeded in doing that. We did not find any dose that was too high to limit proceeding to Phase III. It’s time to proceed with Phase III.”
Schrag stressed that the whistleblowers did not find evidence of manipulated data in the report from the Phase II trial. But given the errors and alleged data manipulation in Zlokovic’s earlier work, he said, it’s appropriate to scrutinize a clinical trial that would administer the product of his research to people in life-threatening situations.
In the Phase II data, “there’s a coherent pattern of [patient] outcomes trending in the wrong direction. There’s a signal in early mortality … there’s a trend toward worse disability numbers” for patients who received the drug instead of a placebo, he said.
None are “conclusive proof of harm,” he said. But “when you’re seeing a red flag or a trend in the clinical trial, I would tend to give that more weight in the setting of serious ethical concerns around the pre-clinical data.”
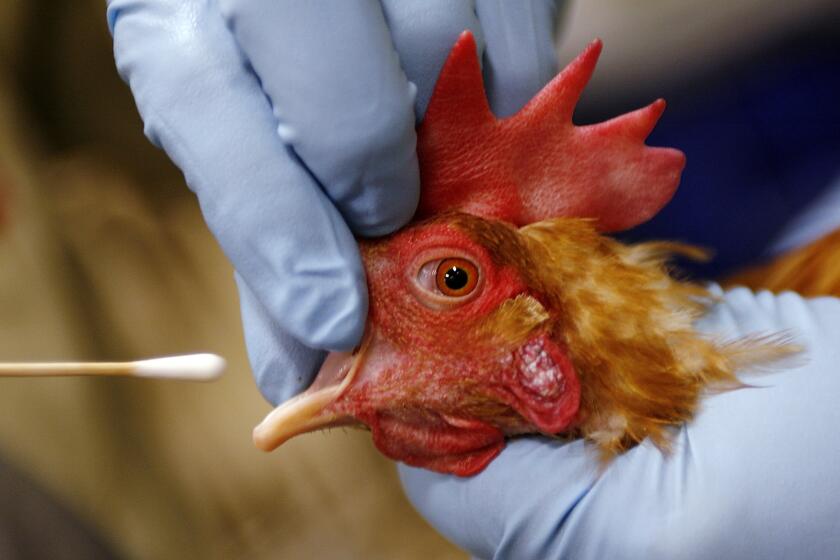
Climate & Environment
What you need to know about the bird flu outbreak, concerns about raw milk, and more
Answering the basics on Bird Flu 2024
May 15, 2024
The NIH paused the clinical trial in November, and it remains on hold, said Dr. Pooja Khatr, principal investigator of the NIH StrokeNet National Coordinating Center. Khatr declined to comment on the pause or the trial’s future, referring further questions to USC and NIH.
The NIH Office of Extramural Research declined to discuss Rhapsody or Zlokovic, citing confidentiality regarding grant deliberations.
ZZ Biotech Chief Executive Kent Pryor, who in 2022 called the drug “a potential game-changer,” said he had no comment or information on the halted trial.
Zlokovic is a leading researcher on the blood-brain barrier, with particular interest in its role in stroke and dementia. He received his medical degree and doctorate in physiology at the University of Belgrade and joined the faculty at USC’s Keck School of Medicine after several fellowships in London. A polyglot and amateur opera singer , Zlokovic left USC and spent 11 years at the University of Rochester before returning in 2011 . He was appointed director of USC’s Zilkha Neurogenetic Institute the following year.
A USC spokesperson confirmed that Zlokovic has retained his titles as department chair and director of the Zilkha institute.
About this article
Corinne Purtill is a science and medicine reporter for the Los Angeles Times. Her writing on science and human behavior has appeared in the New Yorker, the New York Times, Time Magazine, the BBC, Quartz and elsewhere. Before joining The Times, she worked as the senior London correspondent for GlobalPost (now PRI) and as a reporter and assignment editor at the Cambodia Daily in Phnom Penh. She is a native of Southern California and a graduate of Stanford University.
More From the Los Angeles Times
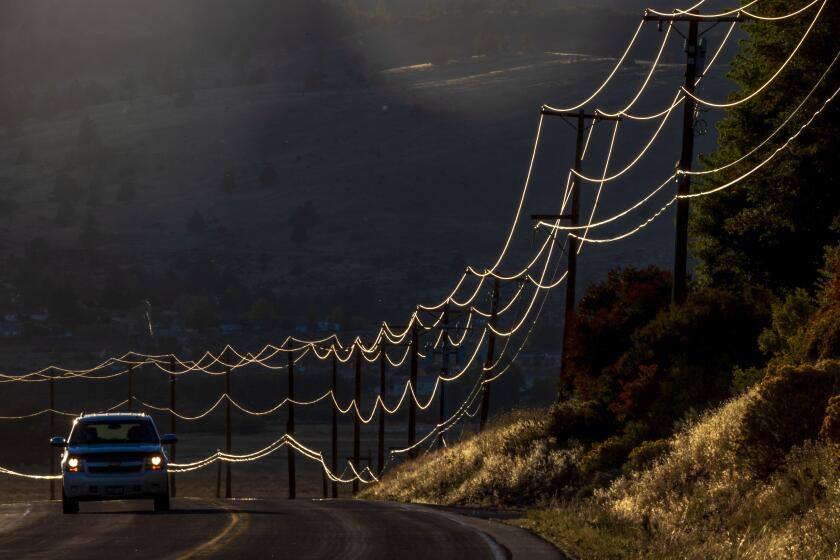
California is changing how big power companies charge for electricity. What to expect on your bill
May 16, 2024
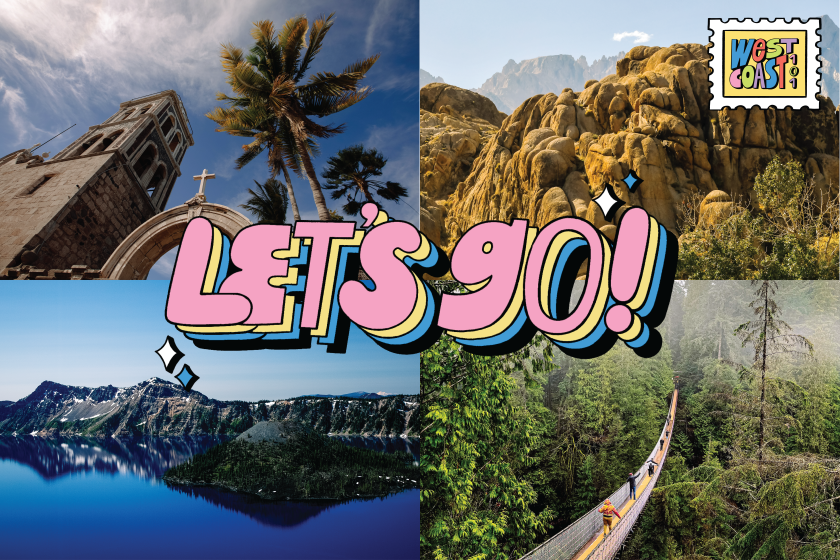
Travel & Experiences
The 101 best West Coast experiences
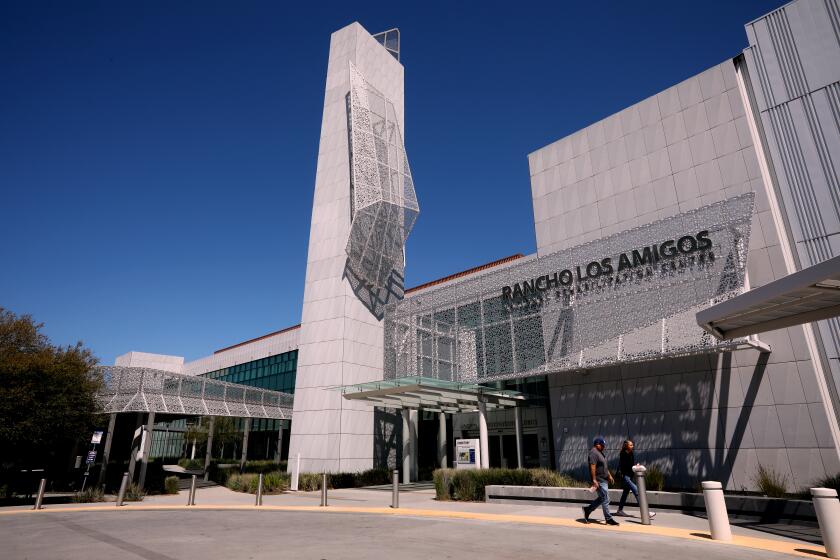
This anesthesiologist is L.A. County’s highest paid employee. He works 94 hours a week
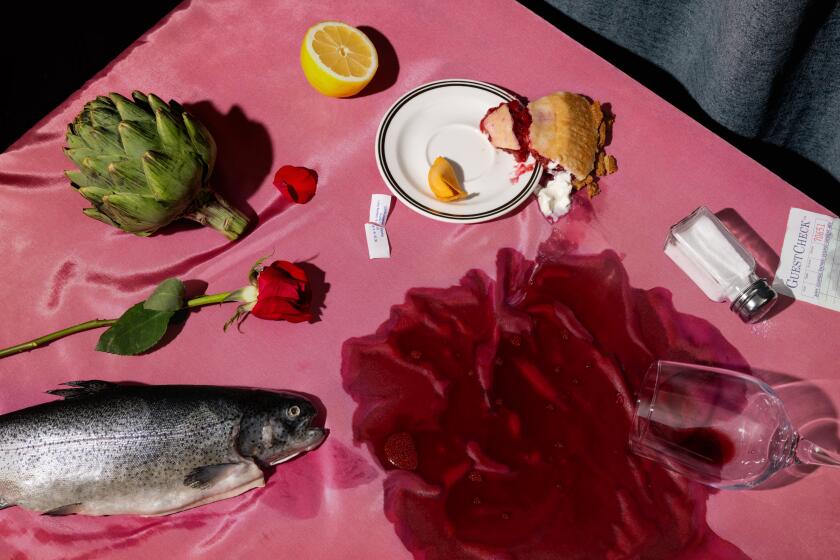
The shocking state of the restaurant industry: ‘We can’t afford to be open. We can’t afford to be closed.’

An official website of the United States government
The .gov means it’s official. Federal government websites often end in .gov or .mil. Before sharing sensitive information, make sure you’re on a federal government site.
The site is secure. The https:// ensures that you are connecting to the official website and that any information you provide is encrypted and transmitted securely.
- Publications
- Account settings
Preview improvements coming to the PMC website in October 2024. Learn More or Try it out now .
- Advanced Search
- Journal List
- Nanomaterials (Basel)

Nanoparticles in Drug Delivery: From History to Therapeutic Applications
Obaid afzal.
1 Department of Pharmaceutical Chemistry, College of Pharmacy, Prince Sattam Bin Abdulaziz University, Al Kharj 11942, Saudi Arabia
Abdulmalik S. A. Altamimi
Muhammad shahid nadeem.
2 Department of Biochemistry, Faculty of Science, King Abdulaziz University, Jeddah 21589, Saudi Arabia
Sami I. Alzarea
3 Department of Pharmacology, College of Pharmacy, Jouf University, Sakaka 72341, Saudi Arabia
Waleed Hassan Almalki
4 Department of Pharmacology, College of Pharmacy, Umm Al-Qura University, Makkah 21955, Saudi Arabia
5 Institute of Molecular Biology and Biotechnology (IMBB), The University of Lahore, Lahore 54000, Pakistan
Bismillah Mubeen
Bibi nazia murtaza.
6 Department of Zoology, Abbottabad University of Science and Technology (AUST), Abbottabad 22310, Pakistan
Saima Iftikhar
7 School of Biological Sciences, University of Punjab, Lahore 54000, Pakistan
8 Department of Pharmacy, COMSATS University, Abbottabad 22020, Pakistan
Imran Kazmi
Associated data.
Not applicable.
Current research into the role of engineered nanoparticles in drug delivery systems (DDSs) for medical purposes has developed numerous fascinating nanocarriers. This paper reviews the various conventionally used and current used carriage system to deliver drugs. Due to numerous drawbacks of conventional DDSs, nanocarriers have gained immense interest. Nanocarriers like polymeric nanoparticles, mesoporous nanoparticles, nanomaterials, carbon nanotubes, dendrimers, liposomes, metallic nanoparticles, nanomedicine, and engineered nanomaterials are used as carriage systems for targeted delivery at specific sites of affected areas in the body. Nanomedicine has rapidly grown to treat certain diseases like brain cancer, lung cancer, breast cancer, cardiovascular diseases, and many others. These nanomedicines can improve drug bioavailability and drug absorption time, reduce release time, eliminate drug aggregation, and enhance drug solubility in the blood. Nanomedicine has introduced a new era for drug carriage by refining the therapeutic directories of the energetic pharmaceutical elements engineered within nanoparticles. In this context, the vital information on engineered nanoparticles was reviewed and conferred towards the role in drug carriage systems to treat many ailments. All these nanocarriers were tested in vitro and in vivo. In the coming years, nanomedicines can improve human health more effectively by adding more advanced techniques into the drug delivery system.
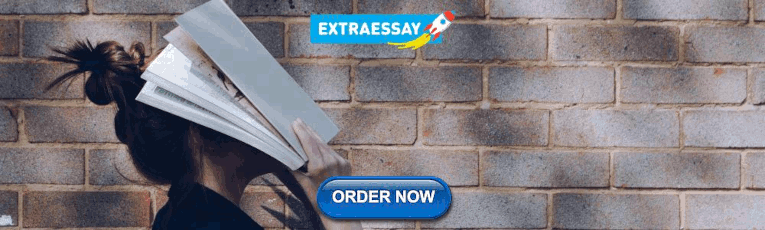
1. Introduction
Drug delivery systems (DDSs) have been used in past eras to treat numerous ailments. All medicines rely on pharmacologic active metabolites (drugs) to treat diseases [ 1 ]. Some of the drugs are designed as the inactive precursor, but they become active when transformed in the body [ 2 ]. Their effectiveness depends on the route of administration. In conventional drug delivery systems (CDDSs), drugs were delivered usually via oral, nasal, inhaled, mucosal, and shot methods [ 3 ]. The conventionally delivered drugs were absorbed less, distributed randomly, damaged unaffected areas, were excreted early, and took a prolonged time to cure the disease [ 4 ]. They were less effective due to many hurdles like their enzymatic degradation or disparity in pH, many mucosal barriers, and off-the-mark effects, and their immediate release enhanced toxicity in blood [ 5 ].
Due to all such reasons, the controlled-release drug delivery system was developed. Such evolution in the DDS enhances drug effectiveness in many ways [ 6 ]. DDSs have been engineered in recent years to control drug release [ 7 ]. Such engineered DDSs used various novel strategies for controlled drug release into the diseased areas. These strategies were erodible material, degradable material, matrix, hydrogel, osmotic pump, and reservoir [ 8 ]. They all provided a medium for the medicines to deliver at the desired sites like tissues, cells, or organs. In these approaches, drugs are often available for many diseases [ 9 ]. Such strategies were unsuccessful due to lower distribution, less solubility, higher drug aggregation, less target selection, and poor effects for disease treatment [ 10 ]. Moreover, drug development is the most expensive, intricate, and time-consuming process [ 5 ]. The innovative drug findings involved the identification of new chemical entities (NCEs), [ 11 ] having the vital distinguishing characteristics of drug capacity and pharmaceutical chemistry. This methodology, however, was confirmed to be less effective in terms of the overall attainment percentage [ 12 ], as 40% of drug development was botched due to its changeable responses and unpredicted noxiousness in humans [ 13 ]. From past decades until now, drug development and its delivery are shifting from the micro to the nano level to prolong life expectancy by revolutionizing drug delivery systems ( Figure 1 ) [ 14 ].

Illustration of how traditional medications were administered without the use of nanocarriers and harm was done to healthy organs or cells. In contrast, modern procedures use nanomedicines to transport medications to specific parts of the body.
In 1959, Feynman was the first physicist to introduce the notion of nanotechnology in the lecture entitled “There’s Plenty of oom at the Bottom”. This concept initiated remarkable developments in the arena of nanotechnology [ 15 ]. Nanotechnology is the study of extremely tiny things and is basically the hub of all science disciplines including physics, chemistry, biology, engineering, information technology, electronics, and material science [ 16 ]. The structures measured with nanotechnology range from 1–100 nm at the nanoscale level [ 17 ]. Nanoparticles have different material characteristics because of submicroscopic size and also provide practical implementations in a wide range of fields including engineering, drug delivery, nanomedicine, environmental indemnification, and catalysis, as well as target diseases such as melanoma and cardiovascular diseases (CVD), skin diseases, liver diseases, and many others [ 18 ].
Therefore, medicines linked with nanotechnology can enhance efficiency of medicines and their bioavailability [ 19 ]. The relation of nanoparticles to biomedicine was demonstrated in late the 1970s, and over 10,000 publications have referred to this association with the term “nanomedicine”. Almost thirty papers on this term were accessible by 2005 [ 20 ].
After 10 to 12 years, Web of Science published more than 1000 nanomedicine articles in 2015 and most of the articles relating nanoparticles (NPs) for biomedical usage [ 21 ]. Nanocarriers such as dendrimers, liposomes, peptide-based nanoparticles, carbon nano tubes, quantum dots, polymer-based nanoparticles, inorganic vectors, lipid-based nanoparticles, hybrid NPs, and metal nanoparticles are the advanced forms of NPs [ 22 ]. Nanoparticles are nowadays a growing arena for drug delivery, microfluidics, biosensors, microarrays, and tissue micro-engineering for the specialized treatment of diseases [ 23 , 24 , 25 ].
Nanoparticles are less effective and can treat cancer by selectively killing all cancerous cells [ 26 ]. In 2015, the Food and Drug Administration (FDA) approved the clinical trials of onivyde nanomedicine in the treatment of cancer [ 27 ]. The characteristic properties of nanocarriers are physicochemical properties, supporting the drugs by improving solubility, degradation, clearance, targeting, theranostics, and combination therapy [ 28 ]. Studies on nanomedicine based on protein used for drug delivery in which various protein subunits combine to deliver medicine on site to a specific tumor have been reported [ 29 ]. Many altered kinds and forms of nanocarriers arranged to carry medicine are protein-based podiums, counting several protein coops, nanoparticles, hydrogels, films, microspheres, tiny rods, and minipellets [ 30 ]. All proteins, including ferritin–protein coop, the small heat shock protein (sHsp) cage, plant-derived viral capsids, albumin, soy and whey protein, collagen, and gelatin-implemented proteins are characterized for drug carriage [ 31 ].
The nanomedicines are escorted in a new-fangled epoch, meant for drug carriage by refining the therapeutic directories of the energetic pharmacological elements engineered inside nanoparticles [ 32 ]. In this epoch, nanomedicine-based targeted-design structures can deliver multipurpose freight with favorable pharmacokinetics and capitalized so as to enhance drug specificity, usefulness, and safety, as shown in ( Figure 2 ) [ 33 ]. The failure of chemotherapeutic approaches has increased the recurrence chances of disease, which enhances the complexity of lethal diseases [ 34 ].

Aids of using nanomedicine platform for delivering drugs to the tumor complex.
Petros and his colleague reported a study about mid-19th century work on nanotechnology. As they reported, polymers and drugs were conjugated in 1955 [ 35 ], the first controlled-release polymer device appeared in 1964, the liposome was discovered by Bangham in 1965, albumin-based NPs were reported in 1972, liposome-based drugs were formulated in 1973, the first micelle was formulated and approved in 1983, the FDA approved the first controlled formulation in 1989, and first polyethylene glycol (PEG) conjugated with protein entered the market in 1990 [ 36 ]. Further studies have produced incredibly encouraging results for treating a variety of disorders ( Table 1 ).
Evolution of nanoparticles from 1991 to 2022 in detail discussed here.
3. Recent Approaches Used in Drug Carriage System for Treatment of Various Diseases
3.1. brain drug delivery system and its types.
Under the most pathological circumstances of diseases such as strokes, seizures, multiple sclerosis, AIDS, diabetes, glioma, Alzheimer’s disease, and Parkinson’s disease, the blood–brain barrier (BBB) is disrupted [ 103 ]. An important reason for the breakdown of the blood–brain barrier is the remodeling of the protein complex in intra-endothelial junctions under the pathological conditions [ 104 ]. Normally, the blood–brain barrier acts to maintain blood–brain homeostasis by preventing entry of macromolecules and micromolecules from the blood [ 105 ]. If a drug crosses the BBB, it restricts accumulation of the drug in the intracerebral region of brain, and bioavailability is reduced, due to which brain diseases cannot be treated [ 106 ]. Therefore, the optimal drug delivery system (DDS) is a cell membrane DDS, virus-based DDS, or exosome-based DDS designed for BBB penetrability, lesion-targeting ability, and standard safety [ 107 ]. For the cure of brain diseases, the nanocarrier-assisted intranasal drug carriage system is widely used [ 108 ]. Now, at the advanced level, drugs poorly distributed to the brain can be loaded into a nanocarrier-based system, which would interact well with the endothelial micro vessel cells at the BBB and nasal mucosa to increase drug absorption time and the olfactory nerve fibers to stimulate straight nose-to-brain delivery [ 109 ], thus greater drug absorption in brain parenchyma through the secondary nose-to-blood-to-brain pathway [ 110 ]. The current strategies used are viral vectors, nanoparticles, exosomes, brain permeability enhancers, delivery through active transporters in the BBB, alteration of administration route, nanoparticles for the brain, and imaging/diagnostics under diseased conditions [ 111 ].
3.1.1. Role of Nanocarriers in Alzheimer’s Disease
Alzheimer’s disease is one of the fastest growing neurodegenerative diseases in the elderly population. Clinically, it is categorized by abstraction, damage to verbal access, and diminishing in spatial skills and reasoning [ 112 ]. Furthermore, engrossment of amyloid β (Aβ) aggregation and anxiety in the brain have significant parts [ 113 ]. The treatment of different diseases with nanotechnology-based drug delivery uses nanotechnology-based approaches [ 114 ]. In Alzheimer’s diseases, polymeric nanoparticles, liposomes, solid lipid nanoparticles, nano-emulsions, micro-emulsions, and liquid-crystals are used for treatment.
Polymeric Nanoparticles
- I. The drug Tacrine was loaded on polymeric nanoparticles and administered through an intravenous route. It enhanced the concentration of tacrine inside the brain and also reduced the whole-dose quantity [ 115 ].
- II. Rivastigmine drug was loaded on polymeric nanoparticles and administered through an intravenous route. It enhanced learning and memory capacities [ 116 ].
Solid Lipid Nanoparticles (SLNPs)
SLNPs enhanced drug retention in the brain area, raising absorption across the BBB [ 117 ]. Some of the drug’s effects are listed below.
- I. Piperine drug is loaded on solid lipid nanoparticles through an intraperitoneal route inside the brain to decrease plaques and masses and to increase AChE enzyme activity [ 118 ].
- II. Huperzine A improved cognitive functions. No main irritation was detected in rat skin when the drug was loaded on SLNPs in an in vitro study [ 119 ].
In recent reports, the coating of SLNPs with polysorbate enhances drug bioavailability [ 120 , 121 ]. Some of the coated NPs are listed below.
- I. The drug clozapine was loaded on a Dynasan 116 [Tripalmitin] lipid matrix coated with surfactant Poloxamer 188, Epikuron 200 to unload the drug safely into the brain microenvironment [ 122 , 123 ].
- II. Vitamin A was loaded on a lipid matrix Glyceryl behenate with coated surfactant hydroxypropyl distarch to unload the drug safely across the BBB [ 124 , 125 ].
- III. Diminazine was loaded on a stearic acid matrix coated with polysorbate 80 to deliver to an infected area safely [ 126 , 127 ].
- IV. Doxorubicin was loaded on stearic acid SLNs coated with Taurodeoxycholate surfactant to deliver the drug without reducing its effectiveness [ 128 , 129 ].
Liposomes have gained attention as auspicious tactics for brain-targeted drug delivery [ 130 ]. The recorded beneficial features of liposomes are their capacity to integrate and carry a large quantity of drugs and their likelihood to adorn their exterior with diverse ligands [ 131 , 132 ].
- Curcumin–PEG derivative was loaded on liposomes and showed high affinity on senile plaques in an ex vivo experiment. Furthermore, in vitro it demonstrated the ability for Aβ aggregation and was taken inside by the BBB in a rat model [ 133 ].
- Folic acid was loaded on liposomes, administered through an intranasal route and absorbed through the nasal cavity [ 134 ].
Nanoemulsions
- I. Beta-Asarone was loaded on nanoemulsions, administered through an intranasal route, and enhanced bioavailability [ 130 ].
Micro Emulsion
- I. Tacrine was loaded on a microemulsion and improved memory. Such nanoparticles absorbed rapidly via the nose to the brain through an intranasal route [ 135 ].
Liquid Crystals
- I. T. divaricate was loaded on liquid crystals and injected through a transdermal route. It increased permanency of the drug in designs and also increased skin infusion and retention [ 136 ].
3.1.2. Role of Nanocarriers in Parkinson’s Disease (PD)
Parkinson’s disease is considered the second most common neurological ailment, and it faces problems in reliable drug delivery for treatment and diagnosis [ 137 ]. The conventional anti-Parkinson’s drug is Levodopa , but it experiences low bioavailability and deprived transfer to the brain; this is the most thought-provoking problem [ 138 ]. To solve this problem, nanotechnology comes to the fore with insightful solutions to solve this problem. Various nanoparticles like metal nanoparticles, quantum dots, cerium oxide nanoparticles, organic nanoparticles, liposomes, and gene therapy are used in PD treatment [ 139 ]. All these nanoparticles enable drugs to enter through numerous ways across the blood–brain barrier (BBB) [ 140 ]. In the current study, Bhattamisra et al. reported Rotigotine drug loaded on chitosan NPs in human SH-SY5Y neuroblastoma cells and delivered from the nose to the brain in rat model of Parkinson’s disease. A study of the pharmacokinetic data proposed that the intranasal route is the best path for a straight channel of rotigotine to the brain [ 125 ].
Ropinirole (RP)
Ropinirole (RP) is a dopamine agonist used for Parkinson’s treatment. RP-loaded solid lipid nanoparticles (RP-SLNs) with nanostructured lipid carriers (RP-NLCs) comprising hydrogel (RP-SLN-C and RP-NLC-C) formulations are better for oral and topical distribution [ 141 ]. Generally, the results confirmed that lipid nanoparticles and consistent hydrogel formulations can be measured as another carriage methodology for the upgraded oral and topical delivery of RP for the active treatment of PD [ 142 ]. Neurodegenerative pathologies such as AD and PD can be treated with solid lipid nanoparticles, as this permits the drug to cross the BBB and reach the damaged area of the central nervous system [ 143 ].
3.2. Mechanism of Nanoparticles’ Brain Drug Delivery (across BBB)
The NPs are commonly administered via intranasal, intraventricular, intraparenchymal routes. All these routes enabled nanoparticles to cross the BBB due to their small size. When nanoparticles reach the BBB, several mechanisms are used, like receptor-mediated mechanisms, active transport, and passive transport to deliver nanoparticles into the brain. Nanoparticles are small in size, can diffuse passively across the endothelial cells of the BBB, and can interact favorably with brain receptors and recognize ligands for interaction ( Figure 3 ) [ 144 ].

Diagram showing the mechanism of targeted drug delivery across BBB in brain microenvironment. Piperine loaded on SLNPs is injected intraperitonially, across BBB efferently to stop plaque formation. Polymeric nanoparticles are used for Tacrine delivery inside the brain, folic acid are loaded on the liposomes crossing blood–brain barrier to treat Alzheimer’s disease, while nanoemulsions and SLNP are loaded with drugs used to deliver medicines inside the targeted brain area to cure Parkinson’s disease.
3.3. Advantages and Disadvantages of Nanomedicines
When employed for brain illnesses, nanomedicines have both benefits and drawbacks ( Table 2 ).
Advantages and disadvantages of nanomedicine.
4. Nanocarriers Role in Major Cancers
4.1. brain cancer.
Brain malignancy is the most critical disease in the sense of treatment [ 150 ]. Malignancies of the brain are most difficult to treat due to limits imposed by the blood–brain barrier [ 151 ]. The brain microvascular endothelium is present in the BBB and creates barriers that distinguish blood from the neural tissues of the brain [ 152 ]. The BBB prevents the entry of harmful toxins, xenobiotic and other metabolites from entering the brain [ 153 ]. The majority of brain cancers include glioma and glioblastoma. Both of these are among the most lethal forms of brain cancer [ 154 ]. The annual occurrence is 5.26 per 100,000 people or 17,000 new diagnoses each year. The most common treatment is radiation surgery and chemotherapy, usually implemented with with temozolomide (TMZ) [ 155 ]. Nanoparticles have a high potential to treat brain cancer because of their small size in nm, tissue-specific targeting properties, and ease in crossing the BBB [ 156 ] ( Table 3 ).
Various nanoparticles involved in brain cancer treatment in recent era.
4.2. Breast Cancer
Cancer causes major deaths all over the world. Tumors spread due to the proliferation of cells [ 171 ], which invade through the lymphatic system to various parts of the body if they becomes malignant [ 172 ]. According to WHO, the ratio of deaths globally due to cancer is assessed to be 13%, attributing 8.2 million deaths every year [ 173 ]. Breast cancer is the most recorded type of melanoma present in only females, and its severity leads to mortality more often than lung cancer [ 174 ]. In 2012, estimated female breast cancer cases were 1.7 million, with 25% of deaths all over the world [ 175 ]. In a recent study, a report published in the name of Global Cancer Statistics 2020: GLOBOCAN estimates the incidence and mortality worldwide for 36 cancers in 185 countries and provides an update on cancer internationally [ 176 ]. A reported estimate is 19.3 million new cancer cases (18.1 million excluding non-melanoma skin cancer) and almost 10 million cancer deaths (9.9 million without non-melanoma skin cancer) occurring in 2020 worldwide. Female breast cancer has exceeded lung cancer as the most frequently diagnosed cancer, with an estimated 2.3 million new cases (11.7%), followed by lung (11.4%), prostate (7.3%), colorectal (10%), and stomach (5.6%) cancers [ 177 ]. For the effective treatment of breast cancer, surgery, chemotherapy, radiation therapy, hormonal therapy, and targeted therapy are performed [ 178 ]. However, nowadays, nanotechnology has gained interest for breast cancer treatment. Various organic and inorganic nanocarriers are used to deliver drugs to the specific target site [ 179 ]. Nanocarriers enhance the hydrophobicity of the anticancer drugs and promote specific target drug delivery [ 180 ]. Organic nanocarriers include polymeric nanocarriers, liposome nanocarriers, and solid lipid nanocarriers, while inorganic nanocarriers include magnetic nanocarriers, quantum dots, and carbon nanotubes (CNTs); both categories show great results towards treatment of heart diseases ( Table 4 ) [ 181 ]. The mechanism of drug delivery in breast cancer is shown in Figure 4 .

Schematic representation of mechanism of drug letrozol loaded on solid lipid nanoparticles (SLNs) and folic acid coupled to SLNs. The whole carrier was delivered inside the animal rat model to treat effects on breast cancer cell lines. Inside cytoplasm, biodegradation occurred, as well as drug release and caspases’ activation inside nucleus, causing apoptosis.
Nanoparticles’ role in treatment of breast cancer.
4.3. Lung Cancer
Lungs are basically responsible for inhalation [ 194 ]. The lung is composed airways (conveying the air inside and outside of the lungs) and alveoli (gas exchange zones) [ 195 ]. In fact, airways are comparatively tough barriers for particles to enter through, while the barrier along the alveolar wall and the capillaries is relatively fragile in the gas exchange component [ 196 ]. The huge exterior area of the alveoli and deep air blood exchange cause the alveoli to be less healthy when affected by environmental injuries. Such injuries may be the reason for some pulmonary illnesses, including lung malignancy [ 197 ]. Several nanoparticles are now being established for respiratory applications that aim at eliminating the restrictions of orthodox drugs [ 198 ] ( Table 5 ). Nanoparticles aid the cure of many lung diseases, such as asthma, tuberculosis, emphysema, cystic fibrosis, and cancer [ 199 ].
Recent discovered nanoparticle’s role in lung cancer treatment.
5. Drug Delivery Approach in Heart Diseases
Cardiovascular diseases include myocardial infraction (MI) [ 213 ], ischemic impairment, coronary artery disease (CAD), heart arrhythmias, pericardial disease, cardiomyopathy (heart muscle disease), and congenital heart disease [ 214 , 215 ]. All these illnesses are the basic main cause of mortality and morbidity in the world [ 216 ]. Cardiac diseases in humans involve incongruity in the morphogenesis of heart arrangement, functionality, and the healing and periodic shrinkage of cardiac muscles [ 217 , 218 ]. Around 50% of patients suffering from MI die within five years [ 216 ]. The insistence for a novel and effective remedy has brought about progress in direct drug carriage to the heart [ 219 ]. Modern therapeutic approaches have been developed to stop the incidence of heart failure after myocardial infarction [ 220 ]. Liposomes, silica NPs, dendrimers, cerium oxide NPs, micelles, TiO 2 NPs, stents with nano-coatings, microbubbles, and polymer–drug conjugates are used for drug delivery. Magnetic nanoparticles like magnetoliposomes (MLs) are made up of the union of liposomes and magnetic nanoparticles. They are used as magnetic-targeted drug delivery [ 221 ]. The PEGylation of MLs increases their rate of flow in the blood, and pairing of the MLs with antibodies raises the rate of active target to pretentious positions [ 222 ]. Namdari and his co-workers performed experiments in a mice model afflicted with myocardial infraction (MI). Liposomes are used with various modifications and in different ways; they are adapted to load drugs on NPs for efficient delivery inside the cell. Cationic liposomes, perfluorocarbon nanoparticles, polyelectrolyte nanoparticles, and polymeric nanoparticles are the modified forms of nanocarriers [ 223 ] ( Table 6 ).
Different forms of NPs; their experiment studies show its role in treatment of heart diseases.
6. Drug Delivery Approach in Skin Diseases
Skin diseases are follicular and cutaneous. These dermatological diseases are treated nowadays with nanotechnology. Nanoparticle delivery for cutaneous disease treatment is preferred, with minor side effects. The conventionally used creams, gels, and ointments are insufficient for delivering drugs due to low penetration in skin tissues. To address this, polymeric, lipid, and surfactant nanocarriers are used. The polymeric micelles enhance drug penetration into the skin tissue to treat skin cancer. As in this reported study, chitosan polymeric NPs, liposomes, and gold nanoparticles can treat atopic dermatitis by improving drug penetration into the dermal and epidermal layers [ 246 ]. Gold nanoparticles are extremely small in size and can penetrate easily and effectively with very low toxicity and no skin damage. As such, they are used widely in nanocarrier formulations for skin diseases.
7. Drug Delivery Approach in Bone Diseases
Bone diseases includes bone defects due to many pathological factors, such as fracture, trauma, osteoporosis, arthritis, infections, and many other diseases. In fact, bone regeneration as a disease treatment is a very complex process, due to which nanomaterials and biological materials are fused to repair bones effectively. The combination of biomaterial and nanomaterial has reduced bone implantation through the development of bone bioscaffolds [ 247 ].
Mechanism of Drug Delivery
The drugs encapsulated inside the nanoparticle is delivered through blood to the targeted area in the bones. The management of the sending nanoparticles as shown herenin ( Figure 5 ).

Mechanism of nanomedicine delivery in bone diseases.
8. Drug Delivery Approach in Blood Diseases
There are various types of blood diseases, like hemopoietic blood disorder, as well as iron deficiency, leukemia, anemia, hemophilia, platelet diseases, and blood cancer. The conventionally used chemotherapeutic system causes damage to the immune system, with high risk of mortality. Bone marrow transplant is also an expensive and intricate process. For example, thalassemia is treated with deferoxamine, a chelating agent to treat excessive iron in the blood. The siRNA-coated nanocomposite has the inhibitory activity for tumor cells in vivo [ 248 ]. The treatment of blood disorders with nanomedicine is still under investigation.
9. Future Challenges of Nanomedicines
In the field of nanomedicine, there are many innovations which show its importance in clinical and other medical aspects. Many scientists have investigated in their research how nanomedicine is involved in treating malignancies and reducing mortality and morbidity rates. However, there are also future challenges that nanomedicines have been facing until now [ 249 ]. The implementation of nanomedicine in clinical practice will face many issues with insurance companies, regulatory agencies, and the public health sector. Until now, the FDA has not developed any specific regulation for the products containing nanomaterials. Due to a lack of nanomaterial standardization and other safety issues, US agencies, such as the EPA and NIOSH, are giving less funding to these research endeavors.
10. Conclusions
Nanotechnology-based nanomedicine is a diverse field for disease treatment. Nowadays, in every sort of disease, nanotechnology is emerging as the best therapeutic to cure disease. At California University, researchers are developing methods to deliver cardiac stem cells to the heart. They attached nanovesicles that directly target injured tissue to increase the amount of stem cells there. Thus, the involvement of stem cells with nanotechnology will develop many solutions for the disease-based queries in the medical arena. However, nanomedicine and nano drugs deal with many doubts. Irregularities and toxicity and safety valuations will be the topic of development in the future. Nanotechnology will be in high demand. Nowadays, drug-targeted delivery through nanoparticles is catching the attention of pharmaceutical researchers all over the world. Nanomedicine will overcome all the side effects of traditional medicines. This nanoscale technology will be incorporated in the medical system to diagnose, transport therapeutic drugs, and detect cancer growth, according to the National Cancer Institute. Experts are trying to treat SARS-CoV-2 with nanomedicine, as nanoparticles with 10–200 nm size can detect, for site-specific transfer, SARS-CoV-2, exterminate it, and improve the immune system of the body. Nanotechnology could help to combat COVID-19 by stopping viral contamination. Highly accurate nano-based sensors will be made in the future that will quickly recognize the virus and act by spraying to protect frontline doctors and the public. Furthermore, many antiviral disinfectants are being developed through nanobiotechnology to stop virus dissemination. In the future, nanotechnology will evolve to develop drugs with high activity, less toxicity, and sustained release to target tissue. Therefore, personalized medicine and nanomedicine both will be potential therapies to treat COVID-19 successfully, as well as to treat upcoming diseases in future.
Acknowledgments
The authors are thankful to Umm Al-Qura University, Makkah, Saudi Arabia, for supporting this project (Project number 224UQU4310387DSR40).
Funding Statement
The Project was funded by Deanship of Scientific Research at Umm Al-Qura University, and this work was supported by Grant Code (Project Code: 22 UQU4310387DSR40).
Author Contributions
Conceptualization, M.S.N. and I.K.; original draft, O.A., M.S.N., B.M. and O.A.; writing—review and editing, O.A., S.I.A., A.S.A.A., A.T., B.M., B.N.M., S.I. and N.R.; funding acquisition, W.H.A. All authors have read and agreed to the published version of the manuscript.
Data Availability Statement
Conflicts of interest.
The authors declare no conflict of interest.
Publisher’s Note: MDPI stays neutral with regard to jurisdictional claims in published maps and institutional affiliations.

Exploring the mechanism behind drug eruptions in the skin
Although medications can often help patients find a cure or respite from their condition, millions of people worldwide suffer from unpredictable drug toxicities every year. In particular, drug eruptions which manifest through symptoms such as redness, blisters, and itching on the skin, are quite common. Severe drug eruptions can become life-threatening and can have long-lasting consequences. Thus, understanding how and why drug eruptions occur is an important area of research in medical science.
To this end, previous studies have identified specific variants of certain genes as potential causal agents of drug eruptions. Scientists believe that the genes encoding the human leukocyte antigen (HLA), a protein expressed on the surface of leucocytes known to play an important role in the immune system, are involved in the onset of drug eruption. However, current theories cannot explain why HLA-related drug eruptions typically manifest on the skin rather than in multiple organs throughout the body.
To address this knowledge gap, a research team including Lecturer Shigeki Aoki, Kousei Ito, and Akira Kazaoka from the Graduate School of Medical and Pharmaceutical Sciences, Chiba University, conducted an in-depth study on the link between HLA and drug eruptions. Their findings were published in PNAS Nexus on April 2, 2024.
The researchers first conducted a series of experiments on keratinocytes from mice, which are the primary type of cells found in the skin. These keratinocytes were engineered to express a specific variant of the HLA gene called HLA-B*57:01, which specifically bind to the antiviral drug abacavir. Then, they validated these findings in genetically modified mice expressing HLA-B*57:01, that were exposed to abacavir.
The researchers found that HLA-B*57:01 expressing keratinocytes that were exposed to abacavir exhibited endoplasmic reticulum (ER) stress responses, such as immediate release of calcium into the cytosol and elevated expression of heat shock protein 70 (HSP70). They also observed an increased production of cytokines and immune cell migration. Abacavir exposure triggered HLA misfolding in the ER, leading to ER stress. Moreover, the researchers observed that the ER stress could be reduced by using 4-phenylbutyrate (4-PB). By alleviating this stress, they managed to suppress the onset of severe drug eruption symptoms. This newfound knowledge could form the basis for innovative treatment options for management of drug eruptions.
But how does this new information contrast with what was already known about HLA? "HLA molecules are an integral component of our immune system, that typically present foreign antigens to white blood cells, which judge these antigens as self or non-self. In this established role, HLAs are usually secondary players," explains Dr. Aoki. "However, our research highlights a novel function of the HLA molecule within skin cells. We revealed that a specific HLA genotype in keratinocytes can recognize certain drugs as foreign, triggering an endoplasmic reticulum stress response."
Taken together, the findings of this study uncover a new role of HLA proteins in sensing and responding to potential threats in skin cells. Thus, their functions may extend well beyond mere antigen presentation for the immune system. Moreover, considering that the variant of HLA possessed by an individual can be determined through genetic testing, this study could help develop preventive measures and diagnostics against severe adverse drug reactions. According to Dr. Aoki, this is in line with current research directions and trends in medical science. "In 10 years, we anticipate entering the 'whole genome era,' where personalized medicine based on individual genomes will become a standard practice," he comments. He further adds, "Building on the findings of this study, we believe that a comprehensive understanding of the mechanism underlying HLA-dependent adverse drug reactions will enable the delivery of safe medical care, allowing patients to avoid unnecessary suffering due to side effects."
Overall, future investigations in this research area might minimize the occurrence of drug eruptions and save people from potentially fatal adverse drug reactions.
About Dr. Shigeki Aoki Dr. Shigeki Aoki is a lecturer at the Graduate School of Pharmaceutical Sciences, Chiba University, Japan. His research focuses mainly on cancer metabolism and drug toxicity. He has authored multiple papers published in reputed journals. Dr. Aoki is a member of various professional bodies in Japan. He has also received several awards for his research, including the Award for Young Scientists conferred by The Pharmaceutical Society of Japan.
- Pharmacology
- Personalized Medicine
- Pharmaceuticals
- HIV and AIDS
- Biotechnology
- Biotechnology and Bioengineering
- Nocebo - Placebo
- Decade Volcanoes
- Drug addiction
- Drug discovery
- Personalized medicine
- Methamphetamine
Story Source:
Materials provided by Chiba University . Note: Content may be edited for style and length.
Journal Reference :
- Akira Kazaoka, Sota Fujimori, Yushiro Yamada, Tomohiro Shirayanagi, Yuying Gao, Saki Kuwahara, Naoki Sakamoto, Takeshi Susukida, Shigeki Aoki, Kousei Ito. HLA-B*57:01-dependent intracellular stress in keratinocytes triggers dermal hypersensitivity reactions to abacavir . PNAS Nexus , 2024; 3 (4) DOI: 10.1093/pnasnexus/pgae140
Cite This Page :
Explore More
- Robotic 'SuperLimbs' Could Help Moonwalkers
- Solving Quantum Many-Body Problems
- When 'Please' Is More Strategic Than Magic
- Autonomous Drones With Animal-Like 'Brains'
- How Practice Forms New Memory Pathways
- Reversing Brain Damage Caused by Ischemic Stroke
- Earth-Sized Planet Orbiting Ultra-Cool Dwarf
- Robots' Sense of Touch as Fast as Humans?
- Avian Flu Detected in NYC Wild Birds
- Metro-Area Quantum Computer Network Demo
Trending Topics
Strange & offbeat.
Suggestions or feedback?
MIT News | Massachusetts Institute of Technology
- Machine learning
- Social justice
- Black holes
- Classes and programs
Departments
- Aeronautics and Astronautics
- Brain and Cognitive Sciences
- Architecture
- Political Science
- Mechanical Engineering
Centers, Labs, & Programs
- Abdul Latif Jameel Poverty Action Lab (J-PAL)
- Picower Institute for Learning and Memory
- Lincoln Laboratory
- School of Architecture + Planning
- School of Engineering
- School of Humanities, Arts, and Social Sciences
- Sloan School of Management
- School of Science
- MIT Schwarzman College of Computing
New treatment could reverse hair loss caused by an autoimmune skin disease
Press contact :, media download.
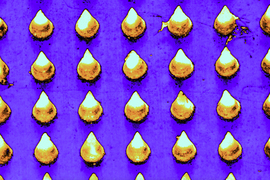
*Terms of Use:
Images for download on the MIT News office website are made available to non-commercial entities, press and the general public under a Creative Commons Attribution Non-Commercial No Derivatives license . You may not alter the images provided, other than to crop them to size. A credit line must be used when reproducing images; if one is not provided below, credit the images to "MIT."
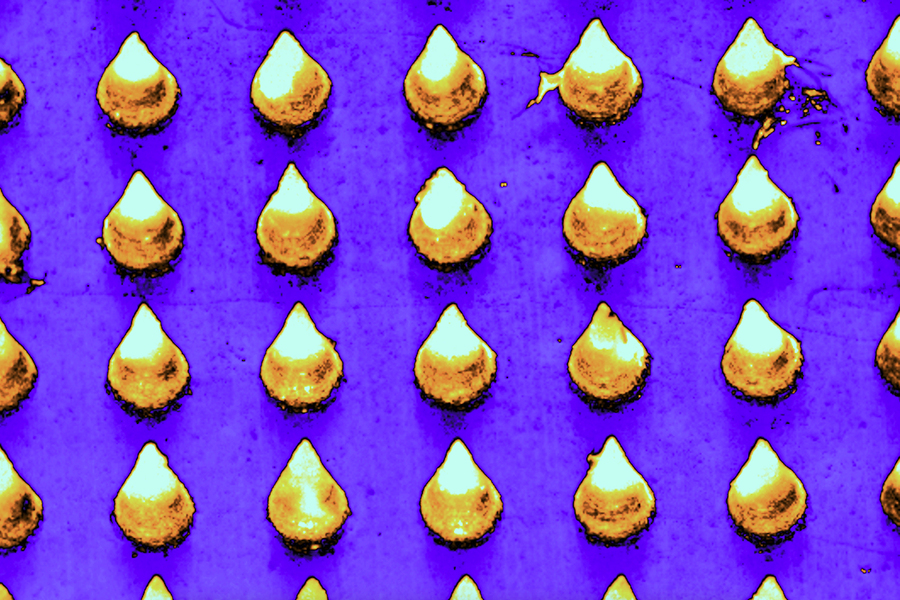
Previous image Next image
Researchers at MIT, Brigham and Women’s Hospital, and Harvard Medical School have developed a potential new treatment for alopecia areata, an autoimmune disorder that causes hair loss and affects people of all ages, including children.
For most patients with this type of hair loss, there is no effective treatment. The team developed a microneedle patch that can be painlessly applied to the scalp and releases drugs that help to rebalance the immune response at the site, halting the autoimmune attack.
In a study of mice, the researchers found that this treatment allowed hair to regrow and dramatically reduced inflammation at the treatment site, while avoiding systemic immune effects elsewhere in the body. This strategy could also be adapted to treat other autoimmune skin diseases such as vitiligo, atopic dermatitis, and psoriasis, the researchers say.
“This innovative approach marks a paradigm shift. Rather than suppressing the immune system, we’re now focusing on regulating it precisely at the site of antigen encounter to generate immune tolerance,” says Natalie Artzi, a principal research scientist in MIT’s Institute for Medical Engineering and Science, an associate professor of medicine at Harvard Medical School and Brigham and Women’s Hospital, and an associate faculty member at the Wyss Institute of Harvard University.
Artzi and Jamil R. Azzi, an associate professor of medicine at Harvard Medical School and Brigham and Women’s Hospital, are the senior authors of the new study , which appears in the journal Advanced Materials . Nour Younis, a Brigham and Women’s postdoc, and Nuria Puigmal, a Brigham and Women’s postdoc and former MIT research affiliate, are the lead authors of the paper.
The researchers are now working on launching a company to further develop the technology, led by Puigmal, who was recently awarded a Harvard Business School Blavatnik Fellowship.
Direct delivery
Alopecia areata, which affects more than 6 million Americans, occurs when the body’s own T cells attack hair follicles, leading the hair to fall out. The only treatment available to most patients — injections of immunosuppressant steroids into the scalp — is painful and patients often can’t tolerate it.
Some patients with alopecia areata and other autoimmune skin diseases can also be treated with immunosuppressant drugs that are given orally, but these drugs lead to widespread suppression of the immune system, which can have adverse side effects.
“This approach silences the entire immune system, offering relief from inflammation symptoms but leading to frequent recurrences. Moreover, it increases susceptibility to infections, cardiovascular diseases, and cancer,” Artzi says.
A few years ago, at a working group meeting in Washington, Artzi happened to be seated next to Azzi (the seating was alphabetical), an immunologist and transplant physican who was seeking new ways to deliver drugs directly to the skin to treat skin-related diseases.
Their conversation led to a new collaboration, and the two labs joined forces to work on a microneedle patch to deliver drugs to the skin. In 2021, they reported that such a patch can be used to prevent rejection following skin transplant. In the new study, they began applying this approach to autoimmune skin disorders.
“The skin is the only organ in our body that we can see and touch, and yet when it comes to drug delivery to the skin, we revert to systemic administration. We saw great potential in utilizing the microneedle patch to reprogram the immune system locally,” Azzi says.
The microneedle patches used in this study are made from hyaluronic acid crosslinked with polyethylene glycol (PEG), both of which are biocompatible and commonly used in medical applications. With this delivery method, drugs can pass through the tough outer layer of the epidermis, which can’t be penetrated by creams applied to the skin.
“This polymer formulation allows us to create highly durable needles capable of effectively penetrating the skin. Additionally, it gives us the flexibility to incorporate any desired drug,” Artzi says. For this study, the researchers loaded the patches with a combination of the cytokines IL-2 and CCL-22. Together, these immune molecules help to recruit regulatory T cells, which proliferate and help to tamp down inflammation. These cells also help the immune system learn to recognize that hair follicles are not foreign antigens, so that it will stop attacking them.
Hair regrowth
The researchers found that mice treated with this patch every other day for three weeks had many more regulatory T cells present at the site, along with a reduction in inflammation. Hair was able to regrow at those sites, and this growth was maintained for several weeks after the treatment ended. In these mice, there were no changes in the levels of regulatory T cells in the spleen or lymph nodes, suggesting that the treatment affected only the site where the patch was applied.
In another set of experiments, the researchers grafted human skin onto mice with a humanized immune system. In these mice, the microneedle treatment also induced proliferation of regulatory T cells and a reduction in inflammation.
The researchers designed the microneedle patches so that after releasing their drug payload, they can also collect samples that could be used to monitor the progress of the treatment. Hyaluronic acid causes the needles to swell about tenfold after entering the skin, which allows them to absorb interstitial fluid containing biomolecules and immune cells from the skin.
Following patch removal, researchers can analyze samples to measure levels of regulatory T cells and inflammation markers. This could prove valuable for monitoring future patients who may undergo this treatment.
The researchers now plan to further develop this approach for treating alopecia, and to expand into other autoimmune skin diseases.
The research was funded by the Ignite Fund and Shark Tank Fund awards from the Department of Medicine at Brigham and Women’s Hospital.
Share this news article on:
Press mentions, healthday news.
MIT researchers have developed microneedle patches that are capable of restoring hair growth in alopecia areata patients, reports Ernie Mundell for HealthDay . The team’s approach includes a, “patch containing myriad microneedles that is applied to the scalp,” writes Mundell. “It releases drugs to reset the immune system so it stops attacking follicles.”
Previous item Next item
Related Links
- Natalie Artzi
- Institute for Medical Engineering and Science
Related Topics
- Drug delivery
- Health sciences and technology
- Institute for Medical Engineering and Science (IMES)
Related Articles
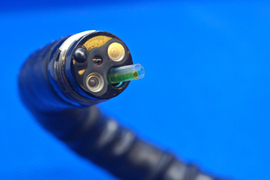
A sprayable gel could make minimally invasive surgeries simpler and safer
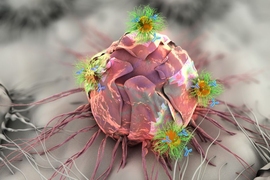
Patch that delivers drug, gene, and light-based therapy to tumor sites shows promising results
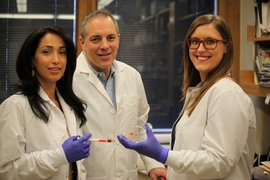
MIT researchers design tailored tissue adhesives
More mit news.
Elaine Liu: Charging ahead
Read full story →
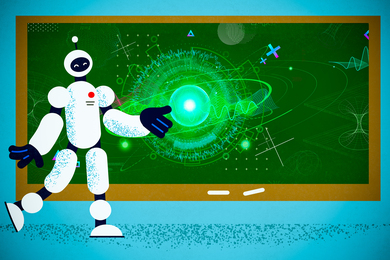
Scientists use generative AI to answer complex questions in physics
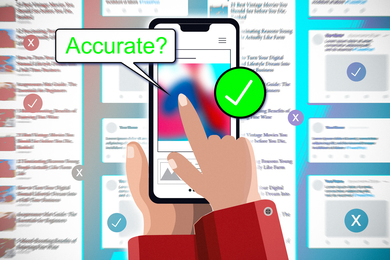
New tool empowers users to fight online misinformation
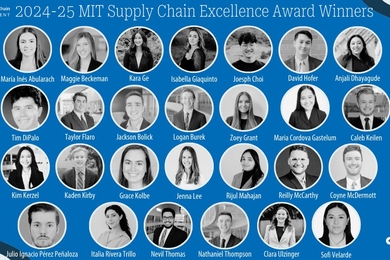
2024 MIT Supply Chain Excellence Awards given to 35 undergraduates
Faces of MIT: Reimi Hicks
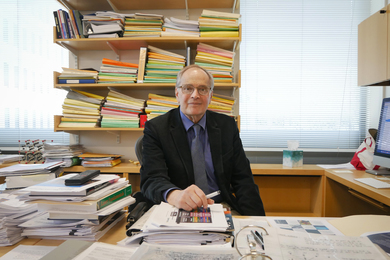
John Joannopoulos receives 2024-2025 Killian Award
- More news on MIT News homepage →
Massachusetts Institute of Technology 77 Massachusetts Avenue, Cambridge, MA, USA
- Map (opens in new window)
- Events (opens in new window)
- People (opens in new window)
- Careers (opens in new window)
- Accessibility
- Social Media Hub
- MIT on Facebook
- MIT on YouTube
- MIT on Instagram
share this!
May 14, 2024
This article has been reviewed according to Science X's editorial process and policies . Editors have highlighted the following attributes while ensuring the content's credibility:
fact-checked
peer-reviewed publication
trusted source
Machine learning model uncovers new drug design opportunities
by Los Alamos National Laboratory
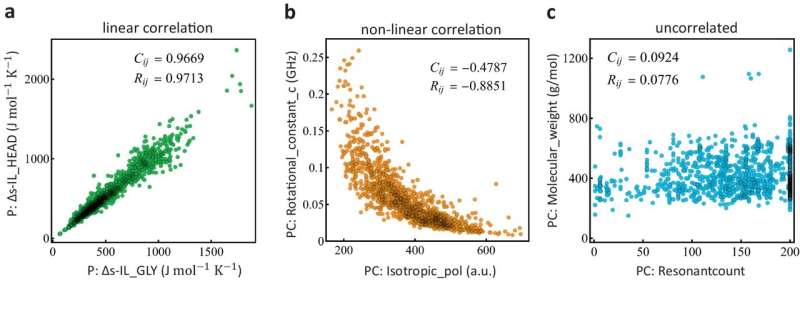
Pathogens are nothing if not adaptable, and their ability to protect themselves against antibiotics increasingly poses a public health concern. A research team led by Los Alamos National Laboratory has used machine learning, an application of artificial intelligence, to identify molecular properties that could guide the discovery of new types of antibiotics, especially among pathogens deemed critical by the World Health Organization due to their high bacterial resistance.
The findings are published in the journal Communications Chemistry .
"Some pathogens have properties that make them very effective at resisting antibiotics," said Gnana Gnanakaran, scientist at Los Alamos. "The discovery of specific compounds able to permeate and inhibit some pathogens is a needle-in-the-haystack challenge due to the vast heterogeneity and depth of the chemical space, and the complexity of the molecular interactions across bacterial membranes. The approach we employ is capable of probing the bacterium-specific, molecular-level profiles necessary that can be built on for successful drug development."
Bacterial defenses against antibiotics
Gram-negative bacteria have an outer membrane less permeable to being breached by compounds, such as those that make up antibiotics, and the bacteria can also expel compounds that happen to get inside, curtailing the effectiveness of an antibiotic.
Data-driven models have potential to identify molecular properties that could overcome such bacterial defenses, but accurate calculations to make those determinations are challenging and use extensive computing resources. Chemically diverse compounds may contain many relevant properties; the machine learning-driven study reduced the relevant spectrum of those properties and established empirical rules that would predict the compound's ability to permeate the bacteria's outer membrane .
Machine learning model identifies pathogen-fighting properties
Focusing specifically on the gram-negative bacteria Pseudomonas aeruginosa, the research team developed a machine learning model to identify the relevant descriptors associated with compounds and predict those compounds' success in permeating bacteria's outer membranes and avoiding expulsion. The team relied on high-performance computing capabilities at Los Alamos to extract the molecular properties of permeation from simulations that considered 1,260 chemically diverse compounds as they traverse the bacterial membrane.
Their analysis sheds new light on the key properties drug candidates need to effectively permeate Pseudomonas aeruginosa, and opens the gate to similar data-driven studies in other gram-negative pathogens.
"The machine learning techniques we've employed in this analysis point to a promising approach for similar data-driven studies in other biological membranes , including the blood-brain barrier ," Gnanakaran said.
Journal information: Communications Chemistry
Provided by Los Alamos National Laboratory
Explore further
Feedback to editors

Researchers discover new family of bacteria with high pharmaceutical potential
37 minutes ago

A golden layer unlocks sharper imaging and faster scanning with X-rays
5 hours ago

From roots to resilience: Investigating the vital role of microbes in coastal plant health
16 hours ago

Temperature, time and blueberry wine: Researchers examine fermentation's effects on health-promoting compounds

Heating proteins to body temperature reveals new drug targets
17 hours ago

What fire ants can teach us about making better self-healing materials

Robotic 'superlimbs' could help moonwalkers recover from falls

A novel multifunctional catalyst turns methane into valuable hydrocarbons
18 hours ago

NASA's Juno provides high-definition views of Europa's icy shell

New research addresses alleged benefits of a vegan diet for dogs
Relevant physicsforums posts, most dangerous chemicals.
13 hours ago
Potassium Iodide as a catalyst for Hydrogen Peroxide
Very confused about naunyn definition of acid and base.
May 15, 2024
Ideas for a project in computational chemistry?
Why don't hydrogen ions have osmotic activity in living organisms.
May 6, 2024
Can you eat the Periodic Table?
Apr 23, 2024
More from Chemistry
Related Stories

Team discovers rules for breaking into Pseudomonas
Nov 22, 2023

Study shows that antibiotics targeting the same enzyme elicit varied responses
Mar 15, 2024

When an antibiotic fails: Scientists are using AI to target 'sleeper' bacteria
Apr 8, 2024

Combined treatment using lipid nanoparticles shows promise against antibiotic resistant bacteria
Nov 7, 2023

Examining how the superbug Pseudomonas aeruginosa reacts to antibiotics
Nov 29, 2021

Dozens of potential new antibiotics discovered with free online app
Nov 18, 2019
Recommended for you

First direct imaging of radioactive cesium atoms in environmental samples
19 hours ago

Researchers identify drug compounds that can reduce prion protein levels in infected cells
20 hours ago

New research employs shutter speed analogies to validate 55-year-old theory about chemical reaction rates
23 hours ago
Let us know if there is a problem with our content
Use this form if you have come across a typo, inaccuracy or would like to send an edit request for the content on this page. For general inquiries, please use our contact form . For general feedback, use the public comments section below (please adhere to guidelines ).
Please select the most appropriate category to facilitate processing of your request
Thank you for taking time to provide your feedback to the editors.
Your feedback is important to us. However, we do not guarantee individual replies due to the high volume of messages.
E-mail the story
Your email address is used only to let the recipient know who sent the email. Neither your address nor the recipient's address will be used for any other purpose. The information you enter will appear in your e-mail message and is not retained by Phys.org in any form.
Newsletter sign up
Get weekly and/or daily updates delivered to your inbox. You can unsubscribe at any time and we'll never share your details to third parties.
More information Privacy policy
Donate and enjoy an ad-free experience
We keep our content available to everyone. Consider supporting Science X's mission by getting a premium account.
E-mail newsletter

Impact of the use of cannabis as a medicine in pregnancy, on the unborn child: a systematic review and meta-analysis protocol
- Find this author on Google Scholar
- Find this author on PubMed
- Search for this author on this site
- For correspondence: [email protected]
- Info/History
- Preview PDF
Introduction: The use of cannabis for medicinal purposes is on the rise. As more people place their trust in the safety of prescribed alternative plant-based medicine and find it easily accessible, there is a growing concern that pregnant women may be increasingly using cannabis for medicinal purposes to manage their pregnancy symptoms and other health conditions. The aim of this review is to investigate the use of cannabis for medicinal purposes during pregnancy, describe the characteristics of the demographic population, and to measure the impact on the unborn child and up to twelve months postpartum. Methods and analyses: Research on pregnant women who use cannabis for medicinal purposes only and infants up to one year after birth who experienced in utero exposure to cannabis for medicinal purposes will be included in this review. Reviews, randomised controlled trials, case control, cross-sectional and cohort studies, that have been peer reviewed and published between 1996 and April 2024 as a primary research paper that investigates prenatal use of cannabis for medicinal purposes on foetal, perinatal, and neonatal outcomes, will be selected for review. Excluding cover editorials, letters, commentaries, protocols, conference papers and book chapters. Effects of illicit drugs use, alcohol misuse and nicotine exposure on neonate outcome will be controlled by excluding studies reporting on the concomitant use of such substances with cannabis for medicinal purposes during pregnancy. All titles and abstracts will be reviewed independently and in duplicate by at least two researchers. Records will be excluded based on title and abstract screening as well as publication type. Where initial disagreement exists between reviewers regarding the inclusion of a study, team members will review disputed articles status until consensus is gained. Selected studies will then be assessed by at least two independent researchers for risk bias assessment using validated tools. Data will be extracted and analysed following a systematic review and meta-analysis methodology. The statistical analysis will combine three or more outcomes that are reported in a consistent manner. The systematic review and meta-analysis will follow the PRISMA guidelines to facilitate transparent reporting [1].
Competing Interest Statement
The authors have declared no competing interest.
Funding Statement
This study did not receive any funding.
Author Declarations
I confirm all relevant ethical guidelines have been followed, and any necessary IRB and/or ethics committee approvals have been obtained.
The details of the IRB/oversight body that provided approval or exemption for the research described are given below:
The study will use ONLY openly available human data from studies published in biomedical and scientific journals.
I confirm that all necessary patient/participant consent has been obtained and the appropriate institutional forms have been archived, and that any patient/participant/sample identifiers included were not known to anyone (e.g., hospital staff, patients or participants themselves) outside the research group so cannot be used to identify individuals.
I understand that all clinical trials and any other prospective interventional studies must be registered with an ICMJE-approved registry, such as ClinicalTrials.gov. I confirm that any such study reported in the manuscript has been registered and the trial registration ID is provided (note: if posting a prospective study registered retrospectively, please provide a statement in the trial ID field explaining why the study was not registered in advance).
I have followed all appropriate research reporting guidelines, such as any relevant EQUATOR Network research reporting checklist(s) and other pertinent material, if applicable.
Data Availability
All data produced in the present work are contained in the manuscript.
View the discussion thread.
Thank you for your interest in spreading the word about medRxiv.
NOTE: Your email address is requested solely to identify you as the sender of this article.

Citation Manager Formats
- EndNote (tagged)
- EndNote 8 (xml)
- RefWorks Tagged
- Ref Manager
- Tweet Widget
- Facebook Like
- Google Plus One
- Addiction Medicine (323)
- Allergy and Immunology (627)
- Anesthesia (163)
- Cardiovascular Medicine (2367)
- Dentistry and Oral Medicine (288)
- Dermatology (206)
- Emergency Medicine (379)
- Endocrinology (including Diabetes Mellitus and Metabolic Disease) (835)
- Epidemiology (11765)
- Forensic Medicine (10)
- Gastroenterology (702)
- Genetic and Genomic Medicine (3731)
- Geriatric Medicine (348)
- Health Economics (633)
- Health Informatics (2392)
- Health Policy (929)
- Health Systems and Quality Improvement (896)
- Hematology (340)
- HIV/AIDS (780)
- Infectious Diseases (except HIV/AIDS) (13303)
- Intensive Care and Critical Care Medicine (767)
- Medical Education (365)
- Medical Ethics (104)
- Nephrology (398)
- Neurology (3493)
- Nursing (198)
- Nutrition (523)
- Obstetrics and Gynecology (673)
- Occupational and Environmental Health (662)
- Oncology (1819)
- Ophthalmology (535)
- Orthopedics (218)
- Otolaryngology (287)
- Pain Medicine (232)
- Palliative Medicine (66)
- Pathology (446)
- Pediatrics (1032)
- Pharmacology and Therapeutics (426)
- Primary Care Research (420)
- Psychiatry and Clinical Psychology (3172)
- Public and Global Health (6135)
- Radiology and Imaging (1279)
- Rehabilitation Medicine and Physical Therapy (746)
- Respiratory Medicine (825)
- Rheumatology (379)
- Sexual and Reproductive Health (372)
- Sports Medicine (322)
- Surgery (401)
- Toxicology (50)
- Transplantation (172)
- Urology (145)
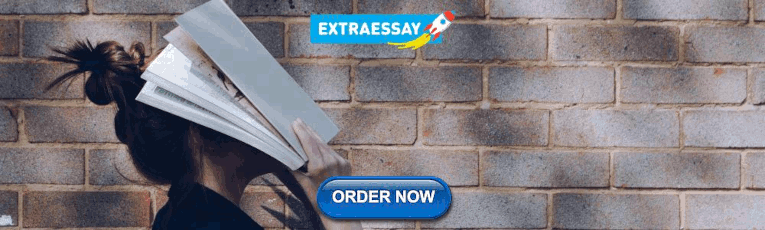
IMAGES
VIDEO
COMMENTS
Nature Reviews Drug Discovery is a journal for people interested in drug discovery and development. It features reviews, news, analysis and research highlights.
The New England Journal of Medicine (NEJM) is a weekly general medical journal that publishes new medical research and review articles, and editorial opinion on a wide variety of topics of ...
Most often, the development of a new medicine starts when basic scientists learn of a biological target (e.g., a receptor, enzyme, protein, gene, etc.) that is involved in a biological process thought to be dysfunctional in patients with a disease such as Alzheimer's disease (AD). Here, we are considering the discovery and development of ...
Drug discovery is the process through which potential new therapeutic entities are identified, using a combination of computational, experimental, translational, and clinical models (see, e.g., [1,2]).Despite advances in biotechnology and understanding of biological systems, drug discovery is still a lengthy, costly, difficult, and inefficient process with a high attrition rate of new ...
About the journal. Medicine in Drug Discovery is a peer-reviewed open access journal. The journal publishes original papers related to basic, preclinical and clinical studies covering all aspects of drug discovery and development. Clinical studies with a focus on efficacy and safety, target engagement, biomarkers, …. View full aims & scope.
Read about the latest advances in translational and clinical research, selected by the Nature Medicine editorial team. Nature Medicine is a Transformative Journal; authors can publish using the ...
Drug design is a complex pharmaceutical science with a long history. Many achievements have been made in the field of drug design since the end of 19th century, when Emil Fisher suggested that the drug-receptor interaction resembles the key and lock interplay. Gradually, drug design has been transformed into a coherent and well-organized ...
Section A-Research paper. Eur. Chem. Bull. 2023,12(Special I ssue 12), 2068 - 2074. 2069. ... New drugs serving unmet medical needs are one of the key value drivers of research-based ...
Research conducted by Raschka et al. [14-15] demonstrates how machine learning can be integrated into G-protein coupled receptor (GPCR) ligand recognition, which is a key part of the drug discovery process. The aim of their research was to determine whether machine learning could replace the old technology used in Sea Lamprey Receptor 1 (SLOR1 ...
The drug discovery landscape has undergone a significant transformation over the past decade, owing to research endeavors in a wide range of areas leading to strategies for pursuing new drug targets and the emergence of novel drug modalities. NMR spectroscopy has been a technology of fundamental importance to these research pursuits and has seen its use expanded both within and outside of ...
Applies for Individuals only. Drug Research (formerly Arzneimittelforschung) is an international peer-reviewed journal with expedited processing times presenting the very latest research results related to novel and established drug molecules and the evaluation of new drug development. A key focus of the publication is translational medicine ...
The numbers for substance use disorders are large, and we need to pay attention to them. Data from the 2018 National Survey on Drug Use and Health suggest that, over the preceding year, 20.3 million people age 12 or older had substance use disorders, and 14.8 million of these cases were attributed to alcohol.When considering other substances, the report estimated that 4.4 million individuals ...
Nanomedicine and nano delivery systems are a relatively new but rapidly developing science where materials in the nanoscale range are employed to serve as means of diagnostic tools or to deliver therapeutic agents to specific targeted sites in a controlled manner. Nanotechnology offers multiple benefits in treating chronic human diseases by site-specific, and target-oriented delivery of ...
This paper presents a birds-eye on a comprehensive overview of the recommendation system and identifies possible research avenues associated with drug repository and ADR. Furthermore, this paper presents clinical post-pre-processing, clinical entity recognition, tweet pooling, and expert rating framework to evaluate the classifier's performance.
We aimed to identify blister packages by their images, photographed using a camera from different angles. In collecting the training set, 72 images were taken for each side of each type of drug: the camera focused from 9 different angles, with 8 different rotation directions of the drug shown in the images (Fig. 2).Both front-side and back-side images were taken for each drug, resulting in a ...
Organ-on-a-chip technology is attracting growing interest across various domains as a crucial platform for drug screening and testing and is set to play a significant role in precision medicine research. Lymph nodes, being intricately structured organs essential for the body's adaptive immune responses to antigens and foreign particles, are pivotal in assessing the immunotoxicity of novel ...
School of Sciences, IGNOU, New Delhi, India. Medicinal plants: Future source of new drugs. Arvind Kumar Shakya. Abstract. India has a long history and strong base for Ayurveda, w hich is the ...
Major AlphaFold upgrade offers boost for drug discovery. Latest version of the AI models how proteins interact with other molecules — but DeepMind restricts access to the tool. Ewen Callaway ...
Research presented by postdoctoral researcher Eda Çiftci, PhD, of the AO Research Institute Davos in Davos, Switzerland, and collaborators during the poster sessions at OARSI 2024 suggest that ...
USC's Berislav Zlokovic has faced questions about the integrity of his research. Since a whistleblower report last year, several papers have been retracted and a drug trial has been paused.
Abstract. Current research into the role of engineered nanoparticles in drug delivery systems (DDSs) for medical purposes has developed numerous fascinating nanocarriers. This paper reviews the various conventionally used and current used carriage system to deliver drugs. Due to numerous drawbacks of conventional DDSs, nanocarriers have gained ...
Pruritus is a side effect of phospho-drugs, but the underlying mechanisms are unclear. Chien et al. screened a drug library for agonists of the human Mas-related G protein-coupled receptor X4 (MRGPRX4) linked to itch and showed that many of the identified drugs had a phosphate group. Because mice do not express MRGPRX4, the authors created a transgenic mouse expressing the human receptor in ...
Thus, understanding how and why drug eruptions occur is an important area of research in medical science. To this end, previous studies have identified specific variants of certain genes as ...
Caption: The microneedle patches used in this study are made from hyaluronic acid crosslinked with polyethylene glycol (PEG), both of which are biocompatible and commonly used in medical applications. The researchers designed the microneedle patches so that after releasing their drug payload, they can also collect samples that could be used to monitor the progress of the treatment.
Machine learning model uncovers new drug design opportunities. by Los Alamos National Laboratory. Basic relationships among the descriptors. Each data point represents a molecule, and it is ...
A research field is being developed to produce nanoparticles and nanotechnology-based treatments. 6.4. Drug delivery. Nanotechnology-based drug delivery systems initially include nanoparticles that contain one or more therapeutic drugs that can bind or scatter and adsorbed polymer matrices.
St. Jude Children's Research Hospital. St. Jude Children's Research Hospital is leading the way the world understands, treats and cures childhood cancer, sickle cell disease, and other life-threatening disorders. It is the only National Cancer Institute-designated Comprehensive Cancer Center devoted solely to children. Treatments developed at St. Jude have helped push the overall childhood ...
Reviews, randomised controlled trials, case control, cross-sectional and cohort studies, that have been peer reviewed and published between 1996 and April 2024 as a primary research paper that investigates prenatal use of cannabis for medicinal purposes on foetal, perinatal, and neonatal outcomes, will be selected for review.
This paper presents the implementation of a Generic Medicine Recommender System (GMRS) that incorporates user feedback as a solution to streamline the selection process of appropriate generic ...