- Open access
- Published: 03 April 2020
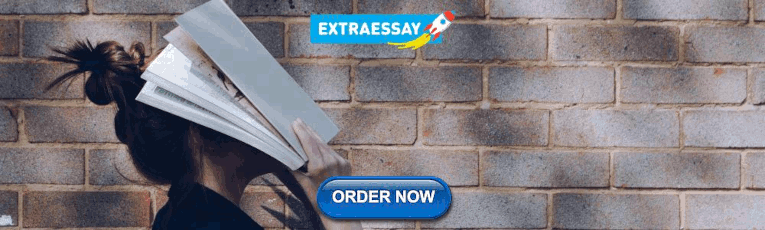
Review on natural products databases: where to find data in 2020
- Maria Sorokina ORCID: orcid.org/0000-0001-9359-7149 1 &
- Christoph Steinbeck ORCID: orcid.org/0000-0001-6966-0814 1
Journal of Cheminformatics volume 12 , Article number: 20 ( 2020 ) Cite this article
75k Accesses
232 Citations
44 Altmetric
Metrics details
Natural products (NPs) have been the centre of attention of the scientific community in the last decencies and the interest around them continues to grow incessantly. As a consequence, in the last 20 years, there was a rapid multiplication of various databases and collections as generalistic or thematic resources for NP information. In this review, we establish a complete overview of these resources, and the numbers are overwhelming: over 120 different NP databases and collections were published and re-used since 2000. 98 of them are still somehow accessible and only 50 are open access. The latter include not only databases but also big collections of NPs published as supplementary material in scientific publications and collections that were backed up in the ZINC database for commercially-available compounds. Some databases, even published relatively recently are already not accessible anymore, which leads to a dramatic loss of data on NPs. The data sources are presented in this manuscript, together with the comparison of the content of open ones. With this review, we also compiled the open-access natural compounds in one single dataset a COlleCtion of Open NatUral producTs (COCONUT), which is available on Zenodo and contains structures and sparse annotations for over 400,000 non-redundant NPs, which makes it the biggest open collection of NPs available to this date.
Introduction
Natural products (NPs), are broadly defined as chemicals produced by living organisms. More precise definitions of NPs exist, but they do not always meet a consensus: some of the NPs include all small molecules that result from metabolic reactions, others classify as “NP” only products of secondary, or non-essential, metabolism. In this review, we made the choice to exclude molecules that participate in the primary, or essential, metabolism, such as energy or anabolic pathways, and consider only molecules that are produced by living organisms in order to accomplish a “higher” function, such as signalling or defence and still smaller than 1500 Da. However, as for most of the definitions in life sciences, the line between primary and secondary metabolites is very thin and depends on the potential application of the molecule to categorise This categorisation justifies the necessity of dedicated NP databases or a proper annotation in generalistic databases of molecules.
NPs have evolved over millions of years and acquired a unique chemical diversity, which consequently results in the diversity of their biological activities and drug-like properties. Therefore, even before the rise of the modern chemical pharmacology, NPs have been used for centuries as components of traditional medicines, in particular as active components of herbal remedies. Nowadays, some of the traditional healing practices, such as Indian Ayurveda, traditional Chinese medicine or African herbal medicines, remain the primary treatment option for many people across the world, due to economic reasons, to personal beliefs or to the difficulty in accessing pharmaceutical products. In modern pharmacology too, NPs have become one of the most important resources for developing new lead compounds and scaffolds [ 1 , 2 , 3 ]. Every week, scientific articles in peer-reviewed journals are published describing the positive effects of NPs on the healing process of various human and animal diseases. Major classes of antibiotics and antifungals are based on NPs isolated from microorganisms. Drugs used in the treatment of various cancers, cardiovascular diseases, diabetes, and more are often NPs or their derivatives. For instance, between 1981 and 2014 over 50% of newly developed drugs were developed from NPs [ 1 ]. NPs and their derivatives are also actively studied in food [ 4 , 5 , 6 , 7 , 8 ], cosmetic industries [ 9 , 10 ] and in agriculture, with natural pesticides development [ 11 ]. This growing interest over NPs and their application resolved in uncontrollable growth of the number of published open and commercial databases, industrial catalogues, books of NPs and collections of structures provided in supplementary materials or research articles, compiling NPs from various organisms, geographical locations, targeted diseases and traditional uses. It became, therefore, a real challenge to find a complete and comprehensive open database for NPs. One other major problem is the publication of structures only in graphical format, such as in the annual reviews of Marine Natural Products [ 2 ]: these are not easily retrievable to be computationally analysed and they are not automatically integrated into public molecular databases. Virtual NP collections are therefore required for virtual screening, which is the first step in all exploratory molecular analyses and to some extent, in the discovery of NP-based drug or other types of active components. For example, the prior virtual screening of known NPs can prevent loss of time with extracting and purifying samples, postponing the wet lab step to the moment of theoretical identification of best candidates. In this way, the usage of modern cheminformatics technologies allows to accelerate research and save time and money for better results. The previous reviews on NPs databases are either outdated and do not reference the actual state of NP resources [ 12 , 13 ], either focus on one particular type of application for such databases [ 14 , 15 ], in particular databases that can be used for dereplication [ 16 ], a particular geographic origin of NPs [ 17 ] or simply do not refer a significant part of NP resources [ 18 ].
For this article, we reviewed a total of 123 resources listing NP structures cited in the scientific literature after 2000. Among them 92 are open and only 50 contain molecular structures that we could retrieve for analyses of their content, the overlap between them and compilation. The quality of molecular structures stored in these databases is also challenging: stereochemistry, for example, plays a major role in the function of NPs, and is the centre of a lot of research projects in the field. Despite this known importance, almost 12% of the collected molecules lack information on stereochemistry while having stereocenters. Finally, the non-redundant collection of NPs from these open resources has been assembled in a MongoDB COlleCtion of Open Natural prodUcTs (COCONUT).
Natural products online resources: availability and characteristics
For now, there is no globally accepted community resource for NPs, where their structures and annotations can be submitted, edited and queried by a large public, like there is UniProt [ 19 ] for proteins or NCBI Taxonomy [ 20 ] for the classification of living organisms. This leads to an impressive (123) amount of various, open and commercial, with different scope and differently structured resources for NP structures and their annotations. Mentions of NP databases, datasets and collections across publications from 2000 to 2019 and in omicX [ 21 ], a catalogue of scientific databases and software, were collected and are listed in Table 1 [ 22 ].
The databases are sorted by alphabetical order of their names and the table lists their various features such as: if they are open or commercial, if they are maintained and updated, what type of NPs they contain and their origin, the approximative number of molecular structures they contain, most recent publication of the collection, if a registration is required to access the data, if extensive metadata is available (taxonomy of the organism producing the NP, tissue, the geographical location where it is isolated, it’s application in (traditional) medicine, diseases it targets, etc.) and if the download of the molecular structures for local use (such as virtual screening) is easy. All these criteria are chosen to evaluate the “FAIRness” [ 23 ] (Findable, Accessible, Interoperable and Reusable) of the NP resources.
For the purpose of this review, the first classification level of the NP databases is their open or commercial access. Next, among the open-access databases, we distinguish databases of metabolites (that contain NPs but also products of primary metabolism), generalistic databases, that do not limit themselves to a particular geographic location or taxonomic classification, databases containing experimental spectra of NPs (NMR, mass spectrometry) and can be used for dereplication applications, thematic databases, that focus on traditional medicine, on drug-like NPs, on the biodiversity of a particular geographic region or on a particular taxonomic group and, finally, open-access industrial catalogues, that are virtual collections of NPs that chemical companies synthesize or isolate and sell. Of course, this segregation is not the only one possible and was made here uniquely for the readability purpose.
Commercial databases
Commercial databases sell the data, access or licence, and in general, it is quite expensive [ 24 ], even for academic use (from 6600 US$ per year for the Dictionary of Natural Products [ 25 ] to over 40,000 US$ for Reaxys [ 26 ] and SciFinder [ 27 ]).
The Chemical Abstracts Service (CAS) launched in 1995 SciFinder [ 27 ], a curated database of chemical information, compiled and maintained by the American Chemical Society. Originally available as desktop software, the web version of SciFinder is available since 2008. As it is CAS that assigns a unique registry number to every chemical substance described in the scientific literature since 1957, the SciFinder contains one, if not the biggest collection of curated chemicals, and, subsequently, of NPs. It is estimated that the number of NPs in SciFinder is over 300,000.
Reaxys [ 26 ] is a database for substances, reactions and documents compiled and maintained by the editor Elsevier. It contains over 10 7 compounds in total, over 200,000 of which are NPs.
The Dictionary of Natural products (DNP) [ 25 ] and it’s autonomous sub-sections, the Dictionary of Marine Natural Products (DNMP) [ 28 ] and the Dictionary of Food Compounds [ 29 ], are the considered as the most complete and best-curated resources for NP.
NaprAlert [ 30 ] was created by researchers at the University of Chicago and contains manually curated information on NPs from literature with rich metadata. Nowadays offers limited free searchers under conditions for academic researchers.
National Institute of Standards and Technology-NIST (version 17) [ 31 ] is one of the standard reference databases for mass spectra (MS) data and is developed and maintained at the National Institute of Health (NIH) in the USA. The main library contains over 250,000 molecules of natural origin (the separation between primary metabolites and NPs is not clearly marked) and is only purchasable on a compact disk.
MarinLit [ 32 , 33 ] is a database of marine NPs based on literature reviews and contain highly curated data that has been collected since the 1970s at the University of Canterbury, New Zealand, and since several years is maintained by the Royal Society of Chemistry (RSC). AntiMarin [ 34 , 35 ] is a historic database of marine NPs that have a described antibiotic activity. While it is still widely cited in thematic studies, the database itself is not accessible anymore, as was apparently merged with MarinLit.
AntiBase [ 36 ] is a comprehensive database of more than 40,000 NPs from microorganisms and higher fungi with very rich metadata collected from literature and manually validated. It is not updated since 2014 and is only available for purchase on Wiley’s website [ 37 ].
eBasis (Bioactive Substances in Food Information Systems) is an online, manually curated collection of 267 foods and 794 active compounds that they contain. The database offers rich and high-quality metadata on food NP activities and structures and limited free access to scientists to try the resource.
The Natural Product Discovery System (NADI) [ 38 ] contains over 3000 natural compounds from more than 15,000 Malaysian plant species. Despite being developed and maintained by the University Sains Malaysia, it is not open for academic use.
ChemTCM [ 39 ] is a database of NPs from plants used in traditional Chinese herbal medicine. The original part of this dataset resides not only in the very rich metadata but also in the predicted activity of NPs against common Western therapeutic targets and their estimated molecular activity according to traditional Chinese herbal medicine categories. The database was developed at King’s College London, in the UK, in part with the support of Innovation China-UK.
The Natural Products Library (NPL) [ 40 ] was described in a paper by AstraZeneca, a famous pharmaceutical company, but the data, containing at the moment of publication over 800 well-curated and annotated NPs, only remained as an in-house collection.
The Ayurveda dataset [ 41 ] was initially a published database of NPs extracted from the Indian traditional medicine plants. The link in the mentioned publication is still working but redirects to a website that provides software solutions for NP and chemistry research in general. Maybe the database is still available together with the software, but the access to it is for subscriptions only.
The Berdy’s Bioactive Natural Products Database [ 42 ] database is mentioned in publications from the 2000s and early beginning of 2010s but is not accessible anymore not even for the purchase of an older version. Originally, Birdy’s company was sending the database as a paper version and with the rise of accessible digital storage, on a digital medium upon order. The company does not seem to exist anymore.
Open-access databases
We could identify a total of 92 open-access NP resources across the literature in the last 20 years. The concept of “Open-access” encourages and prioritizes free and open online access to academic information, such as data and scientific publications. For a dataset, whether in a database or attached as additional information to an article, it means that anyone can read, download, copy, distribute, print, search for and within and re-use all or parts of data that are contained in it. For this review, we have endeavoured to compile an exhaustive list of open-access NP resources that have been cited at least ones in a peer-reviewed scientific publication after the year 2000. As the number of such sources is quite substantial (87), a thematic classification for them has been established. First, we present larger databases of organic molecules that also contain metabolites and NPs. These are followed by the presentation of databases containing molecular spectra (mass spectrometry or NMR) that can be used for the dereplication process for the identification of organic molecules and, in particular, of NPs in experimental data. Next, the scope will be narrowed with databases containing only NPs but without any taxonomic, usage or geographic selection on them. The most diverse data source category is the so-called “thematic” one: it contains databases of NPs that focus on a particular taxonomy (e.g. plants, bacteria, fungi), on a particular usage (e.g. Chinese, Indian or African traditional medicine, NPs found in food or toxic NPs) or on a particular geographic location (e.g. marine NPs, Brazilian and Mexican biodiversity NPs). Finally, are introduced industrial catalogues of NPs. These are made available by chemical companies that synthesize or purify NPs on command.
Databases of metabolites and chemicals
The first starting points in the search for structures for organic molecules are these big chemical libraries. They contain a wide range of organic compounds, and metabolites and NPs are well identifiable in them. The reference libraries, widely accepted by the scientific community as sources of reliable molecular information are: ChEBI [ 43 ], ChEMBL [ 44 ], ChemSpider [ 45 ], PubChem [ 46 ] and ChemBank [ 47 ]. ChEBI is developed and maintained at the European Bioinformatics Institute (EBI) and its main focus is chemical ontologies, i.e. structural relationships between molecules; it contains over 15,000 clearly identified NPs. ChEMBL is also the product of EBI but it has a wider focus and is considered as a repository for experimentally elucidated molecular structures and, in particular, drugs and drug-like chemical; it contains over 1800 NPs, but this number is very probably underestimated because of the unclear labelling of molecules as NP in this database. PubChem is an integrated platform of small molecules and biological activities is an initiative of the US (NIH) and is one of the major sources for biomolecules discovery and submission. It contains over 3500 NPs, although, similarly to ChEMBL, this number is very underestimated due to the unclear labelling of compounds as NPs. ChemSpider is a chemical database offering very rich metadata, cross-references to a lot of other chemical sources and advanced search. It is maintained by the Royal Society of Chemistry and contains over 9700 easily findable NPs. ChemBank was developed by the Broad Institute of Harvard and MIT and was dedicated to the storage of raw screening data of small organic molecules. This resource is unfortunately not available anymore due to maintenance difficulties, although all data remains available for a bulk download, but is not as handy to search.
There are also databases that focus only on metabolites, chemicals that are produced by living organisms (generally, but not only through enzyme-catalyzed reactions) and that are involved in primary and secondary metabolisms. The two major and most comprehensive databases for metabolites covering most of the domains of life are KEGG [ 48 ] and MetaCyc [ 49 ]. They contain an equivalent amount of chemicals, also involved in secondary metabolism, i.e. NPs, but present a different point of view on data organization and have been widely compared in the literature [ 50 ]. The BRENDA database [ 51 ] focuses on enzyme activities, but also contains the compounds involved in enzyme-catalyzed reactions, and this, covering most of all known domains of life. The particularity of this database is the manually validated compounds, reactions and enzyme activities in its main part, and exhaustive taxonomic origins for enzymes and compounds; however, NPs and primary metabolites are not clearly separated in this resource, so it is difficult to estimate their respective numbers. The Chemical Structure Lookup Service (CSLS) [ 52 ] was developed for a very rapid metabolite structure lookup in an aggregated collection of more than 80 databases comprising more than 27 million unique structures in 2007. Not updated anymore, it is still possible to download the datasets, but the lookup service is not available so the extraction of NPs only requires an extensive data curation. The last database presented in this section is BiGG [ 53 ]: a platform for highly-curated genome-scale metabolic models. It contains, as parts of the metabolic models metabolites, but the distinction of primary and secondary metabolism is not clear, so it requires a lot of efforts to extract information on NPs only.
Databases for dereplication
Dereplication is one important step in experimental NP discovery as it prevents re-isolation and re-characterization of already known molecules. It consists of a lookup in databases with annotated experimental data (mainly mass spectrometry (MS) and Nuclear Magnetic Resonance (NMR) spectra) for comparison to newly obtained experimental data, and its annotation in case of found spectral identity. There are two big categories of databases used for dereplication based on the type of spectra they contain, MS and NMR.
Databases for dereplication for MS data
There are three distinct databases called “MassBank”: the MassBank of North America (MoNa) [ 54 ], the European MassBank [ 55 ] and the Japanese MSSJ MassBank [ 56 ]. The three contain reference MS spectra for metabolites and extensive metadata. MoNa tends to be favoured by the scientific community as it integrates data from more sources than the two others, contains rich and community-curated metadata and facilitates the submission of new datasets.
METLIN [ 57 ] is a database that allows the characterization of known metabolites and a technology platform for the identification of known and unknown metabolites and other chemical entities. It is a comprehensive resource containing over 1 million molecules including primary metabolites, toxins, small peptides, and NPs. METLIN’s high-resolution tandem mass spectrometry (MS/MS) database, which plays a key role in the identification process, has data generated from both reference standards and their labelled stable isotope analogues, facilitated by METLIN-guided analysis of isotope-labelled microorganisms. However, it does not allow an easy download of the data, but the access to the platform is free for academic use.
The Human Metabolome Database (HMDB) [ 58 ] is a metabolomic database containing comprehensive information on human metabolites with very extensive metadata and reference spectra. It contains human-produced NPs together with NPs that are essential for the function of the human organism. However, as it is the case in a lot of previously described databases, the separation between NPs and primary metabolites is tricky.
From the same institution, the Yeast Metabolome Database (YMDB) [ 59 ], was created with the same pattern as the HMDB, and therefore also contains very extensive metadata for baker’s yeast metabolites, enzymes that are involved in the molecular metabolism and reference spectra. Again, the separation between NPs and primary metabolites is difficult, do this dataset was not included in further analysis either.
The RIKEN MSn spectral database for phytochemicals (ReSpect) is a collection of in-house and literature MS plant NP spectra. The website is still maintained and is usable but the last dataset has been added in 2013.
The Global Natural Products Social Molecular Networking (GNPS) [ 60 ] is a web-based knowledge base containing MS spectra for NPs only and is intended to be the base for the community-wide organization and sharing of raw, processed or identified data. In addition to providing access to spectra, it is also possible to download solely the structures of the NPs from this database.
Databases for dereplication for NMR data
NMRshiftDB [ 61 ] an open and peer-reviewed database for organic molecules structures and their NMR spectra. It contains a big number of easily identifiable NP spectra that makes it the reference tool for NP dereplication applications.
NMRdata [ 62 ] is a Chinese initiative for the storage and elucidation of NP structures from NMR data. Unfortunately, the main website is in Chinese and the English version is limited. To access the data one needs an account in a university that participates in the NMRdata project. At the moment of the writing of this manuscript, NMRdata contains 1,167,468 spectra, which theoretically makes it the biggest resource for NMR data in the world but it is under-used due to the language barrier.
NAPROC-13 [ 63 ] is a database containing 13C spectral information of over 6000 natural compounds. All data is accessible and searchable online, however, it is not possible to download the subsequent structures.
Spektraris NMR database [ 64 ] is a collection of NMR spectra that are focusing on plant NPs. The more than 400 spectra from more than 200 compounds in this database were manually transcribed from the literature. Spectra from this database are also submitted to NMRshiftDB to profit of the advanced technological aspects of the latter.
Generalistic databases of natural products
Generalistic public databases for NPs are not specialized in any particular type of NP nor on NP origins or usages. They are generally intended as catalogues for various purposes, such as in silico screening for activity prediction, molecular docking and so on. Seven generalistic public NP databases that have been active in the last 20 years have been identified from the literature.
SuperNatural II [ 65 ] is a database that contains over 300,000 NPs together with their 2D structures, computed physicochemical properties and predicted toxicity. It also provides references to the chemical suppliers for the actual purchase of the molecules, but not to other chemical databases. The database is maintained but is probably not updated anymore as some of the companies selling molecules are not active anymore (such as MDPI [ 66 ]). Unfortunately, SuperNatural does not provide a bulk download, even if the download of separate MOL files for molecules is possible and erroneously does not contain only NPs (e.g. it contains dodecahedrane, identified in this database under SN00136231 and it is not a NP), so this resource needs to be used with caution despite its wide fame in the scientific community.
The Universal Natural Products Database (UNPD) [ 67 ] was an effort to compile all know NPs in one collection for in silico drug screening. The last accessible version of the UNPD contains over 200,000 NP structures. The database is not accessible anymore through the link provided in the original publication, but a copy of the molecular structures contained in it is still maintained on the ISDB [ 68 ] website (a database for in silico predicted MS/MS spectra for NPs).
ZINC [ 69 ] is a public access database and toolset that was initially developed to enable easy access to chemical compounds for virtual screening purposes and that became ever widely used for a big range of cheminformatic applications. It has a very clear separation of molecules in catalogues, in particular on their origin, and contains an easily searchable and retrievable collection of over 85,000 NPs.
The Natural Product Activity and Species Source Database (NPASS) [ 70 ] contains over 30,000 NPs from plants, bacteria, fungi and animals and is developed and maintained at the National University of Singapore. This database was created to provide a reliable source for highly curated NPs with structures, experimental activity values and the organisms that synthesize them.
RIKEN Natural Products Encyclopedia (NPEdia) [ 71 ] contains over 25,000 secondary metabolites isolated from various species and annotated with rich metadata, such as molecule origin and physicochemical and biological properties. The database is still available online but is not updated since 2014.
3DMET [ 72 ] is a database that was created in 2005 in the National Institute of Agrobiological Sciences in Japan and is still maintained and updated until now. The idea of such a database came during the conversion from 2D to 3D NP structures and the errors that were occurring during it that needed manual curation. Currently, the database contains over 18,000 entries, cross-referenced to the KEGG database [ 48 ], but unfortunately, the download of the structures is not possible.
The Chinese Natural Products Database (CNPD ) [ 73 ] is a generalistic database created by Chinese researchers in order to facilitate the virtual screening of NPs for drug discovery purposes. This database is mentioned in over 120 papers until 2010 but is impossible to localize, as there is no URL provided in the original publication of the database and the dataset is not added as supplementary information to it. It is therefore probably incorrect to cite this database as a data source for NP, as the only possible sources found (from NeoTrident Technology Ltd) are in Chinese only.
One big negative point is that in ZINC, SuperNatural II and UNPD databases, the three biggest ones in terms of the number of NPs, the taxonomic nor geographic origins of the organism that produced the compound cannot be identified and in general they lack metadata and literature references.
For the completeness of this list, it is also necessary to site two major tools for the discovery and prediction of NPs from protein sequence data: antiSMASH [ 74 ] and PRISM [ 75 ]. Both are trained on, among others, NP data, but the latter is not provided directly to the public.
Thematic databases
Thematic databases for NPs focus on one particular origin or application of these secondary metabolites. Here we list databases that contain NPs produced by a particular domain of life (e.g. plants, fungi, bacteria), produced by organisms living in a particular geographical location (e.g. marine organisms, South American organisms) or by its application (traditional medicines, food or drugs). Apart from some rare exceptions, thematic databases tend to be small (less than 3000 entries) and very specialized.
In order to avoid biological provenance confusion, it needs to be noted that in some cases, NPs isolated from plants and animals can actually be synthesized by microorganisms that live on or in the host [ 76 ]. This is particularly the case of endophytes, bacteria living inside plant cells and very difficult to differentiate from the latter during preparation for metabolomics experiments [ 77 ]. Although the confusion is rare due to the improvement of identification methods and genetic approaches, it can create a bias in reproducibility of the NP isolation and needs, therefore, to be taken into account.
Natural products by the taxonomy of the synthesizing organism
KNApSaCK [ 78 ] is a comprehensive database for plant NPs that contains over 10,000 retrievable 2D and 3D structures, information on the relationships between the NPs and their expressing organism(s). It is pretty difficult to navigate despite the original design choices, and it does not offer a bulk download of the dataset.
Collective Molecular Activities of Useful Plants (CMAUP) [ 79 ], a relatively new database, contains very extensive information on plants that are linked to human activities together with their chemical constituents, i.e. NPs. The database offers very rich metadata for NPs, such as the plants that produce them and their geographical distributions.
TriForC [ 80 ] is a European Union-funded project that aims for the “discovery and production of known and novel bioactive triterpenes for pharmaceutical and agrochemical development”. The database contains a pipeline for triterpenes discovery and 266 NPs together with the enzymes and pathways leading to their production. It contains metadata for the compounds, but no structures in computer-readable format nor the possibility of downloading them.
Alkamid database [ 81 ] references over 300 N -alkylamides from plants, a promising group of bioactive compounds in drug and crops research. The database is fully open and offers rich metadata, in particular, the taxonomical classification of plants that produces the NPs, but does not allow a bulk download of any information from it.
The Tea Metabolome Database (TMDB) [ 5 ] is a curated and literature-based database for tea components. Not accessible anymore, it contained over 1300 constituents found in tea.
Microorganisms
StreptomeDB [ 82 ] is a collection of NPs from bacteria from the Streptomyces genus, which is very important for the production of natural bioactive compounds such as antibiotics, antitumour and immunosuppressant drugs. These bacteria are of particular importance in pharmacological research as around two-thirds of all known natural antibiotics are produced by them. While collecting data for this review, we encountered some difficulties to access the website, but the data was downloadable. In addition, an old dataset is available on ZINC.
The Natural Products Atlas (NP Atlas) [ 83 ] is maintained at the Simon Fraser University in Canada and is curated by a consortium of data curators around the world. It is designed to cover NPs from microbes (bacteria, fungi, lichens and cyanobacteria) published in the peer-reviewed literature. The resource is actively updated, allows a bulk download of all data and metadata and since September 2019 is completely open.
ProCarDB [ 84 ] is a database for carotenoids produced by bacteria. It contains over 300 compounds with rich metadata and structures but does not offer any download option.
PAMDB [ 85 ] is a comprehensive Pseudomonas aeruginosa metabolome database, well-curated, with rich metadata and offering bulk download. However, it does not contain only NPs but also results of the primary metabolism, so it was not included in the COCONUT collection.
The Lichen Database [ 86 ] is a collection of over 200 metabolites that have been isolated and identified experimentally in lichens. The database is not available yet, but the data has been already published in the MetaboLights [ 87 ] repository for metabolomics experimental data.
Natural products by use
- Traditional medicines
The World Health Organization listed between 1999 and 2009 a list of over 21 000 plants used for medicinal purposes all over the world [ 88 , 89 ]. This effort was made for proper identification of safe plants, as it is estimated that plant-based traditional medicines are used by 60% of the world’s population [ 90 ]. In addition to efforts to establish formal, DNA-based identification of such plants for wider use [ 91 ], collections of medicinal plant species, and in particular of phytochemicals, NPs produced by plants, associated to their therapeutic activities and physicochemical properties are being established around the world. This is particularly the case in Asia and Africa, where traditional medicines remain an important part of everyday life for cultural, traditional and economic reasons.
Traditional Chinese Medicine (TCM) is naturally part of the Chinese public health system [ 92 , 93 ]. It is therefore coherent that in this country the scientific study of natural compounds from plants used in TCM is very advanced and is receiving strong governmental support, and they have developed a plethora of databases containing NPs, their sources and effects.
The biggest database containing NPs used in TCM is TCM@Taiwan [ 94 ]. It contains over 58,000 entries and is directly feeding iSMART [ 95 ], an integrated cloud computing web server for online virtual screening, evolution studies and drug design. In addition to this, there are several other, smaller, databases for NPs TCM that can be cited, such as the Chinese Ethnic Minority Traditional Drug Database (CEMTDD) [ 96 ], that is maintained, but not updated and contains 4000 NPs, the Chinese Traditional Medicinal Herbs Database (CHDD) [ 97 ], not maintained anymore, but according to the publication contained over 30,000 entries, now not accessible and probably lost for the scientific community. Some other databases containing phytochemicals and other active compounds used in TCM can be cited, such as the Comprehensive Herbal Medicine Information System for Cancer (CHMIS-C) [ 98 ] that is not maintained anymore, the Encyclopaedia of Traditional Chinese Medicine (ETCM) [ 99 ], that is maintained but the chemical structures it contains are not easily retrievable, the database of medicinal materials and chemical compounds in Northeast Asian TM (TM-MC) [ 100 ], which is maintained, updated, but no structures but contains precise plant species for all compounds, the Traditional Chinese Medicine Integrative Database (TCMID) [ 101 ], maintained, but not updated anymore, The Traditional Chinese Medicine Systems Pharmacology database and analysis platform (TCMSP) [ 102 ], that is also not maintained anymore but used to contain over 29,000 NPs. One can quickly realize that there is a lot of databases that focus on chemical compounds used in TCM, and creators of the latter recognize it: there is even a database called “Yet Another Traditional Chinese Medicine Database” (YaTCM) [ 103 ] that was published in 2018. Mainly, all these databases differ in the number of compounds they cover, in the richness of their metadata and on the availability of the datasets they contain.
Another extremely important traditional medicine in Asia is the Indian Ayurveda, that also got a wide popularization worldwide over the past decade. There are, however, very few databases listing natural compounds from plants, insects and animals used in Ayurveda, and they do not contain as many entries as the Chinese ones. Only two are currently online and open. The first one, IMPPAT [ 104 ] is the manually curated database of over 10,000 phytochemicals extracted from 1700 Indian medicinal plants, their phytochemistry and their therapeutic effects. The other, MedPServer [ 105 ] contains NPs from plants from North-East India used in traditional medicine. It aims towards the understanding of the therapeutic mechanisms of action of the 1124 NPs from these plants by integrating ligand-based and structure-based approaches. NeMedPlant [ 106 ] is a small (over 100 NPs) database of active compounds from plants used in North-East Indian traditional medicine, with rich metadata focused on the plants that produce the compound but without possibilities of downloading any information and is not updated anymore. Because it was cited in several peer-reviewed papers, we also need to mention TIM [ 90 ], the database created in 2011 for the Prediction of Biologically Active Natural Products from Ayurveda Traditional Medicine but never linked to an actual database not listing the NPs in the supplementary material of the publication.
Phytochemica [ 107 ] is a small database of plant-derived chemicals that contains plants from Himalaya used in both Chinese and Indian traditional medicines. There are also some databases of NPs that specialize in traditional medicines of other parts of Asia, such as the Database of Indonesian Medicinal Plants [ 108 ] and TIPdb [ 109 ] for plants from Taiwan, but most of them are relatively small and contain in general only few hundreds of compounds.
African Traditional Medicine (ATM) is the other extremely rich and developed traditional medicine with a lot of modern efforts to study, rationalize and put its teachings to the benefit of modern medicine. As for the CTM and the Ayurveda, it requires inventorying plants used by African traditional doctors, identifying the parts that are used to efficiently cure and then identify the active components that they contain. It exists also a certain number of databases focusing on NPs from plants used in traditional medicines on the African continent. Among those, the most famous and the most generalistic is AfroDB [ 110 ], although it is only accessible through the ZINC catalogues. The pan-African natural products library (p-ANAPL) also needs to be cited here, as it focuses on plants used in ATM and is available as the supplementary information if its publication [ 111 ]. Three datasets, AfroCancer [ 112 ], AfroMalariaDB [ 113 ] and Afrotryp [ 114 ], available as supplementary information of their respective publications link NPs from plants used in traditional medicines to their potential targets involved in the treatment of cancer, malaria and Trypanosoma. There are then country-specific and relatively small databases for NPs extracted from ATM plants, such as the Cameroon Medicinal Natural Products database (CamMedNP) [ 115 ], Central African Medicinal Plants database (ConMedNP) [ 116 ] and the Ethiopian Traditional Medicine Database (ETM-DB) [ 117 ].
Databases of drug-like natural compounds
Not linked, at least directly, to the traditional medicines, there is a lot of pharmacological research around the therapeutic properties of NPs, and these are compiled in the databases for drugs and drug candidates. In these databases, natural compounds are generally associated with a type of disease or molecular targets or receptors they interact with, and a rich description of their molecular and overall effects on the state of a patient or of a healthy person. The reference database in this category is DrugBank [ 118 ]. It latest version, which was greatly modified and curated compared to previous ones, contains over 10,000 drugs, among which 3732 are approved drugs and 200 approved drugs that have been produced by a living organism. In order to select only the latter, one needs to search for “nutraceuticals” in the search bar of the DrugBank website [ 119 ]. The previous version of Drugbank, 4.0 [ 120 ], contained over 8000 nutraceuticals, and they were added to COCONUT.
BindingBD [ 121 ] is an interesting database for pharmaceutical research as it contains measured binding affinities of proteins that are supposedly targets of drugs, with small drug-like molecules. Although it does contain NPs and their protein targets, they are not clearly distinguishable from synthetic drugs in this database.
The Novel Antibiotics Database [ 122 ], that is still surprisingly online, is not updated since 2003 and contains 5430 compounds of natural origin with an antibiotic activity that have been published in the Journal of Antibiotics between 1947 and 2003. However, no structure is available for download, only compound names, their activity and the organisms they were isolated from.
ChemIDplus [ 123 ] is a database part of the TOXicology DataNETwork and chemicals that have a relationship with diseases, environment, environmental health and poisoning. It contains rich metadata for each chemical, including its physicochemical properties but also its impact on health and environment. A simple search for “natural product” returns more than 9000 entries, it is however not possible to bulk download the results of the query.
The Herbal Ingredient Targets (HIT) [ 124 ] and the Herbal Ingredients in vivo Metabolism (HIM) [ 125 ] databases are two inter-connected collections of NPs from mainly (but not only) Chinese plants. Both are not accessible online anymore, but the structures of the NPs they contained are available on ZINC. They contained very extensive metadata on the molecular targets of the herbal active ingredients, their toxicity, a wide range of pharmacologically relevant molecular descriptors and their therapeutic effects. Unfortunately, this metadata is not available on ZINC and is probably lost.
There are several databases that focus on collecting information on NPs with anticancer properties and their mechanisms of action. The first one, NPCARE [ 126 ] contains over 6000 NPs from plants, marine organisms, fungi and bacteria with validated anticancer activities and contains extensive metadata. The website is available and seems updated but cannot be accessed sometimes, probably due to server failures on the maintenance side. The Indian Plant Anticancer Compounds Database (InPACdb) [ 127 ] is not available anymore but used to contain very broad information covering pharmaceutical and physicochemical properties of 144 NPs, cancer types and molecular targets. Fortunately, the data is still available on GitHub [ 128 ]. Another database, containing phytochemicals with anti-cancer properties is the Naturally Occurring Plant-based Anti-cancer Compound-Activity-Target (NPACT) database [ 129 ] is still maintained and accessible It contains 1574 manually curated entries with rich metadata on NPs and their therapeutical mechanisms on different types of cancer. The US National Cancer Institute also maintains and makes freely available a number of small (390 on average) natural compound datasets [ 130 ] that are selected as of interest in anticancer research and are currently undergoing tests in various research groups from the US NIH.
InflamNat [ 131 ] is a small (200 NPs) but well-curated dataset of NPs with anti-inflammatory activity. The dataset consists of NP structures, their type and origin and literature references, and is available as supplementary information for its publication.
BioPhytMol [ 132 ] is a manually curated database of natural compounds from plants that have an antibacterial effect. The database has over 2500 entries with very rich metadata, in particular regarding the plant species from which the compounds were extracted. The database is open and maintained but does not offer a bulk download option to be used to further analyses.
The last database in this section is the Open Source Malaria [ 133 ], which is a very nice project as it is a totally open-source collaborative project for anti-malarial drugs discovery that already encountered certain success [ 134 ]. Drug candidates tested in this project are often of natural origin, but as the focus of this database is to collect their effects, it is not always specified, so the content of OSM was not integrated into COCONUT.
FooDB [ 8 ] is the reference database on chemical food constituents associated with extremely rich and diverse metadata. It is developed by the Wishart research group and supported by the Canadian Institutes of Health Research. In total it contains over 22,000 NPs and offers a convenient bulk download their structures.
BitterDB [ 6 ] collects bitter-tasting natural compounds associated with rich metadata on their receptors. However, it also contains synthetic molecules with a bitter taste, and in this database, it is difficult to separate them from the natural ones.
Phenol-Explorer [ 135 ] is a comprehensive database on polyphenol content in food. It currently contains over 800 phenol structures from over 400 foods. Data is derived from the scientific literature, and all data is associated with rich metadata and is available for download.
PhytoHub [ 136 ] is a database of dietary phytochemicals and the human and animal metabolites that derive from them. Over 1200 NPs from more than 350 foods are available in this resource, together with rich metadata and references to other chemical and spectral databases it, unfortunately, does not offer a bulk download for the moment.
The SuperSweet database [ 4 ] is a collection of various molecules, mainly from plant origin, but also synthetics that have a sweet taste. Their structures together with information on their number of calories, therapeutic uses and sweetness index are available. The database is still maintained but is not updated since 2011 and does not provide a bulk download of its content.
A toxin is a substance that is toxic for one or more living organisms and that has a plant or animal origin. Despite this original definition, more and more resources on toxins also integrate molecules from non-organic origin massively present in the environment as they also have a harmful effect on the living organisms. For instance, Exposome-explorer [ 137 ] is a manually curated database of biomarkers of exposure to environmental and dietary factors, and it also contains these factors and their structures. A lot of the toxic environmental and dietary factors in it are from natural origin, but also, approximately half of the compounds in this database are not NPs, which is reasonable, as, for example, environmental pollution is anthropogenic. In the same way can be mentioned the T3DB [ 138 ], the toxin and toxin-target database, as it contains a number of toxins produced by the living organism but its focus is on synthetic toxins and how human metabolism reacts to them.
The biggest (over a 1000) database of animal toxins was the Animal Toxin Database (ATDB) [ 139 ], designed originally to collect toxin structures, origins and effects, but it is not available anymore at the URL provided in the publication. More specialized databases were also published, such as the International Venom and Toxin Database [ 140 ], the Snake Neurotoxin Database [ 141 ], the Mollusk Toxin Database [ 142 ] or the Scorpion Toxin Database [ 143 ]. Unfortunately, most of these databases were based on unformatted text and were lacking effective systems for data query, and none of them is not accessible anymore. It is also unknown if the data contained in these databases is lost or is still available in some generalistic resources.
The last in this section, the Toxic Plants—Phytotoxins Database (TPPT) [ 144 ], is accessible and is maintained and updated by the Agroscope in Switzerland. It contains over 1500 phytotoxins from Central Europe and offers high-quality metadata and a convenient bulk download.
The two databases described next could not be fitted in any of the previous categories. The Carotenoids database [ 145 ] is a collection of NPs produced by a wide range of organisms and that share common substructures (polyene with possibly terminating rings) and properties as they are all yellow, orange or red pigments. Carotenoids produced by plants have particular importance for the nutritional value of the consumed food [ 146 ], but plants are not the only producers of this molecular type which is demonstrated in the Carotenoids database. This database is developed and maintained at the RIKEN institute. SuperScent [ 10 ] is a database of volatile compounds essential from an organic origin that can be scented by humans and animals. It contains over 2000 compounds with their structures and properties but does not offer any download and most of the compound pages are now working. This database is maintained at Charité Belin but is not updated since 2010.
Natural products by the geographic origin of producing organisms
There is a number of country-level efforts to catalogue the biodiversity of NPs in particular geographical zones, generally defined by country political borders. These databases are mainly plant-focused, but can also integrate NP produced by insects, by microorganisms and animal toxins. In this part, the databases are cited in the geographical order from West to East. The last part is describing collections of NPs from organisms in marine and ocean environments.
BIOFAQUIM [ 147 ] is a database published in 2019 and offers for full download over 400 unique NPs from plants, fungi and propolis from Mexican flora and fauna, the species from which the compounds were extracted and their geographical location. The Nuclei of Bioassays, Ecophysiology and Biosynthesis of Natural Products Database (NUBBEDB) [ 148 ] is the first NP library from Brazilian biodiversity. It currently contains over 2000 NPs, highly curated and good quality metadata and easy download of all or partial data. The UEFS dataset [ 149 ] is a collection of NPs isolated from Brazilian plants and maintained by the State University of Ferriera de Santana in Bahia, Brazil. The NPs in this collection have been published separately but there is no common publication nor public database for it, it is however accessible via ZINC.
Three databases contain NPs from the African flora and fauna. The Northern African Natural Products Database (NANPDB) [ 150 ] contains over 4500 NPs from plants, endophytes, fungi and bacteria. The database provides rich metadata, literature references, cross-references to major chemical databases and an easy bulk download. The South African natural compound database (SANCDB) [ 151 ] is very similar to NANPDB in its quality and contains over 600 NPs isolated from South African biodiversity. It is also possible to submit new molecules and to participate in the curation of the database. The Mitishamba database [ 152 ] contains 1100 NPs isolated from Kenyan plants. The database is still maintained but does not seem to be updated and it is possible to download data from it only by requesting an account.
ChemDB [ 3 ] and MAPS database [ 153 ] are two databases for natural compounds from Pakistani plants. Unfortunately, none of them is accessible anymore. VIETHERB [ 154 ] is a database published in 2018 with the aim of providing high-quality and literature-based data on herbs and active compounds from them. Despite the novelty of the database, it is not accessible anymore.
The oceans cover 71% of the surface of the Earth, therefore databases that collect NPs from marine organisms are expected to be broad, complex and cover a wide range of organisms. Unfortunately, the biggest repositories for marine NP structures are commercial (e.g. MarineLit [ 33 ] and DMNP [ 28 ] presented above). In the marine NP community, the major trend is to publish newly discovered molecules in specialised journals (such as the Journal of Natural Products [ 155 ] or Marine Drugs [ 156 ]) as images and rich textual description that are not, for now, easily machine-retrievable.
In the last 20 years, four databases containing structures of marine NPs and their metadata were published. Two of them are not accessible anymore: the Marine Compound Database (MCDB) [ 157 ] and the Marine Natural Product Database (MNPD) [ 158 ]. Both contained only a few hundreds of entries according to their respective publications but these were comprising rich metadata which is now lost. The Dragon Exploration System on Marine Sponge Compounds Interactions (DESMCI) [ 159 ] is still accessible but seems not to be maintained as the actual data, such as molecular structures and the corresponding metadata is not visible when one tries to access it. The Seaweed Metabolite Database (SWMD) [ 160 ] is the only one really maintained and it contains 1110 entries, with only 423 unique structures. Molecular structures in this database are annotated with the species of the algae that produce them, together with the geographical origin of the latter, biological activity of the compound and its physicochemical properties.
Industrial catalogues
A lot of companies that are synthesizing and isolating chemical compounds offer a catalogue of their products, and in some cases, these catalogues also contain the structures and annotations. These catalogues are often cited in the scientific literature as sources of NP structures, therefore it was important to mention the most used catalogues in this review. Surprisingly, a non-negligible number of cited catalogues of NP structures are accessible only to clients, on-demand or to registered users. This is the case of the NP catalogues from Ambinter-Greenpharma natural compound library [ 161 ], ChemBridge diversity datasets [ 162 ] (their NP catalogue seems to be not available anymore), LOPAC1280 by Merk [ 163 ], Prestwick [ 164 ] and TargetMol [ 165 ]. Open NP catalogues are provided by the following: AnalytiCon Discovery [ 166 ], InterBioScreen [ 167 ], Indofine Chemical Company [ 168 ], Pi Chemicals Systems [ 169 ] and Specs [ 170 ]. The website of the latter is not offering the download of their NPs catalogue anymore, but a dataset is available on ZINC [ 171 ]. Note that only the most famous and cited in academic research are listed and more industrial catalogues for NPs exist.
The biggest problem nowadays is that there are too many sources for NPs. A non-experienced researcher in NPs (and even a more experienced one) will just get lost in this variety and diversity of possible data sources. The next major problem is access to data and its maintenance. Indeed, a lot of publications point to a website that is not maintained anymore. This is the case of the majority of animal toxins databases, but also of a number of small regional or traditional medicine databases. In the list of NP sources presented in Table 1 , over 20% are not maintained anymore or the access is intermittent. In some rare cases, the information on the NP structures is still recoverable via the ZINC database, but it is not the case of more modern databases and ZINC does not store any metadata from these collections, only the molecular structures encoded in SMILES. Also, the description and origins of the NPs (i.e. metadata), in addition to their structure are generally lacking, and it is especially the case in data aggregators that are nevertheless the most commonly used. This leads to cases where in silico screening reveals potentially interesting compounds but requires way more efforts and investigations to identify its origins and the way of obtaining it experimentally. Only 40% of NP databases offer an easy bulk download of molecular structures that they contain for further analyses with local tools. The quality of the molecular structures might also require additional attention and curation efforts. Indeed there are no standards for NP databases for a definition of stereochemistry, aromaticity or isotopes, which leads to a variety of possible versions of the same molecule.
This multiplicity of databases comes also from the publishing pressure on scientists, the infamous “publish or perish”. Nowadays, publishing a dataset or a database is a relatively easy publication and have the potential to generate a high number of citations. However, this trend generates a plethora of databases that are unmaintained beyond the publication time (like it is the case of VIETHERB [ 154 ] for example, published only 1 year prior to the writing of the present review and already not accessible anymore), despite the journals requirements to provide accessibility to the published datasets and databases for a number of years ahead.
Comparison and analysis of the content of open NP databases
The 50 NP collections from which NP structures could be downloaded were analysed in order to evaluate their overlap in terms of molecular structures and coherence of their content. 19 physicochemical properties, such as molecular weight, NP-likeness [ 172 , 173 ], logP, TPSA Efficiency, and Zagreb Index, were computed and their distributions are shown in an interactive graphic at https://npreview.naturalproducts.net . Due to the high number of databases to compare, a non-interactive would not be visible. Globally, the physicochemical properties of all datasets are comparable. The NP subset of Drugbank contains molecules that are less likely to be NPs, which can be explained by its high content in NP-derived drugs and the difficulty in dissociating the latter from synthetic ones. The average mass of all NPs in the assembled collection is of 454 Da, and the Spektraris and TCM@Taiwan databases contain the heaviest molecules: both contain molecules with an average of 612 Da. The logP is a lipophilicity measure commonly used in analytical chemistry; the more it is positive, the more lipophilic is the compound and the more negative, the more hydrophilic. Here, the logP was computed with two algorithms, AlogP and XlogP available in the CDK [ 174 ]. In general, NPs tend to be lipophilic, which allows them to have higher membrane penetration, but all datasets also contain in lesser amounts, hydrophilic molecules. CarotenoidsDB and the SeaWeed Metabolites Database outstand from others with their very lipophilic content. On the other side, ReSpect contains more hydrophilic molecules than other datasets.
The overlap in terms of molecular structures between the databases was also calculated and is presented in Fig. 1 and in Additional file 1 : Table S1. In Fig. 1 , which represents a network of overlap between databases, there is a directed edge between database A and database B if more than 50% of the unique molecules from database A are present in database B. An interactive version of this network, where the user can change the percentage of similarity between databases to display is available at https://npreview.naturalproducts.net . It should be noted that 40 of the 50 open NP databases have an overlap of at least 50% with at least one other open database. Except for the Lichen Database, all datasets share at least 10% of their compounds with at least one other open dataset.
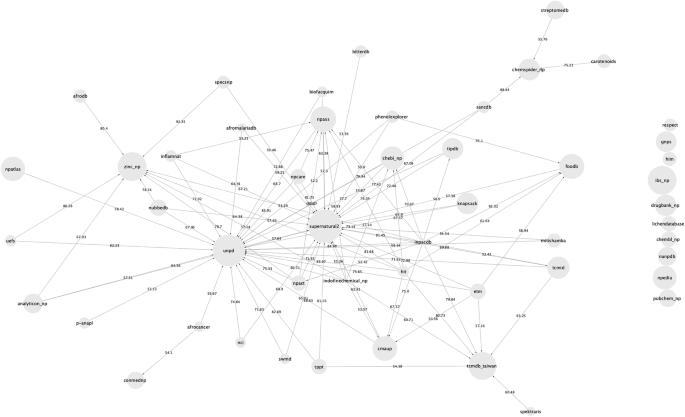
Network of content similarity between the 50 open natural products databases. The network is directed, and there is an arrow from database A to database B if more than 50% of molecules in database A are also present in database B. The interactive version of this network is available at https://npreview.naturalproducts.net
In the majority of the databases, stereochemistry is defined for at least some of their content. Only three databases, TCMid, ReSpect, and NPCARE don’t have any stereochemistry defined for any of the molecules in them. The fraction of NPs with stereochemistry in each database is accessible in Table 1 . On average in the open NP databases, more than 50% of the molecules have a defined stereochemistry. When a 2D molecular structure is present in two databases and stereo information was elucidated, in general, open databases tend to agree on the latter. Doing a pairwise comparison between databases on their overlapping content, pairs of databases tend to agree on the stereochemistry, in on average 70% of NP than they share. The whole list of pairwise agreement between databases on the stereochemistry of their overlapping molecules can be found on FigShare ( https://doi.org/10.6084/m9.figshare.11926047.v2 ).
Five NPs are found in 34 of these 50 databases: apigenin, quercetin, kaemferol, catechin and naringenin. Interestingly, belong all to the flavanol group, part of the flavonoids family and share a common skeleton (Fig. 2 a) with only differences in hydroxy groups. In the top ten most frequent molecules in open databases, in addition to more flavonoids, there is also coumaric acid (Fig. 2 b), gallic acid (Fig. 2 c), scopoletin (Fig. 2 d) and ellagic acid (Fig. 2 e). According to the literature, all these compounds are well-known plant products, however, most of the flavanols, coumaric acid and scopoletin are also present in the bacterial NP database, StreptomeDB.
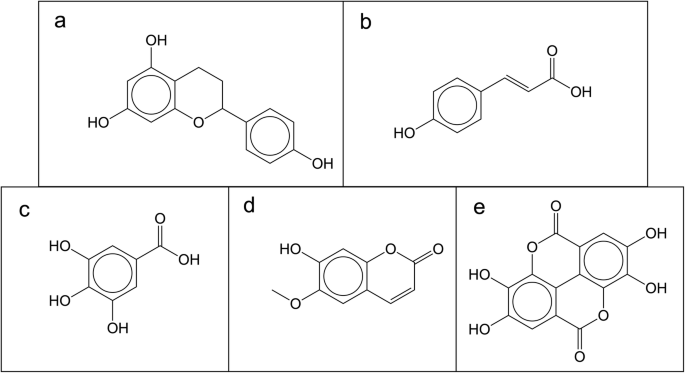
Most frequent molecules in open databases. a Common biggest substructure in the top 5 most frequent molecules, found in 34 out of 50 open databases. b Coumaric acid; c gallic acid; d scopoletin; e ellagic acid
COlleCtion of Open NatUral producTs (COCONUT)
In its current version, COCONUT contains 411,621 unique molecules, unified on the stereochemistry-free InChi keys, that were collected from 50 open and accessible NP databases, listed in Table 1 . This number is big, as this dataset still needs to undergo a curation process, as, despite their claims, some of the NP collections do not contain only natural compounds. 27.9% of molecules in COCONUT do not have stereo centres defined in any of the databases where they have been collected from. Among the latter, 57.7% (66,374 unique molecules) have truly no stereocenters, and the remaining 48,611 NPs have at least one stereocenter, but this information is not provided.
50% of the unique molecules have only one stereochemical version of their 3D structure, and 22.1% have more than one. The latter could be different valid stereoisomers of the same base constitution or errors in the databases. Addressing those errors will be subject of future curation of COCONUT. When a 2D molecule has several possible 3D structures, these can originate from the same public database, where stereochemistry is precisely defined, but also from different databases. Note that unknown NP structures or mixtures are not included in COCONUT. The collection is available as a MongoDB dump and a CSV file on Zenodo ( https://doi.org/10.5281/zenodo.3547718 ) and a user-friendly web interface to browse it is under development. The aim of COCONUT is to make the NP-related data as FAIR as possible.
There are currently 123 data collections of natural products (NPs) that have been published and cited in the scientific literature between 2000 and 2019. Only 50 of them are open access or have their content accessible (in ZINC for example) and among them, the overlap of their content is significant, as 40 of these datasets share at least 50% of the compounds they contain with at least one other dataset.
There are several aggregators, such as the ZINC catalogue for NPs, SuperNatural II and UNPD (not maintained anymore), but they do not cover the entire space of known NPs and do not allow submissions of newly discovered compounds.
There is a need for an aggregator database for NPs, that will be commonly recognized, well organized and allowing an easy submission of newly found molecules, like it is the case for UniProt for proteins.
Conclusions
Natural products are important molecules for medical, chemical and social research. There is no, for now, any universal, community-accepted database for NP discovery, screening and dereplication. Instead, there is an extremely high number of very diverse databases and datasets, not all maintained or open access in 2020, which represents a serious loss of knowledge. There is a need for a unified universal repository for NPs, to avoid the unnecessary duplication of online resources and facilitate NP research. For the purpose of this review, a COlleCtion of Open Natural prodUcTs (COCONUT) has been assembled, analyzed and made available in Zenodo ( https://doi.org/10.5281/zenodo.3547718 ). A web interface is currently under development for user-friendly querying, exploration and download of the known open NP space. In the future, the annotations of the molecules contained in COCONUT will be improved, in particular, systematically linking the compound to the first publication where it was described and to the organisms that synthesize it.
Materials and methods
All databases in Table 1 were downloaded in July and September 2019. Molecular structures were processed with CDK 2.3 and, when available, annotations were parsed with Java (code available on GitHub https://github.com/mSorok/COCONUT ). Resulting original and non-redundant collections of NPs are stored in a MongoDB database, available as a dump on Zenodo ( https://doi.org/10.5281/zenodo.3547718 ). Redundancy was eliminated based on InChi Keys, computed without stereochemistry (JNI-inchi option of the InChi generator set to “Snon”, “ChiralFlagOff” and “AuxNone”). Stereochemistry was not taken into account during this unification step as it is encoded differently between some databases and there are databases where it is not encoded at all. The overlap between databases in terms of similar stereochemistry was also performed with CDK 2.3. All network representations of overlaps between databases are made with Cytoscape [ 175 ]. Plots and comparative analyses made with Python and the Plotly and Dash libraries. The code for the interactive plots is available on GitHub at https://github.com/mSorok/NPDBReviewDash .
Availability of data and materials
Data and software are freely available under the MIT license. The source code for data processing can be freely obtained from GitHub (github.com/mSorok/COCONUT), the COCONUT data is available on Zenodo ( https://doi.org/10.5281/zenodo.3547718 ). The interactive application for natural products exploration is available at https://npreview.naturalproducts.net/ and the code is available on GitHub ( https://github.com/mSorok/NPDBReviewDash ). The table compiling all assembled natural products resources is available on FigShare ( https://doi.org/10.6084/m9.figshare.11926047.v2 ).
Newman DJ, Cragg GM (2016) Natural products as sources of new drugs from 1981 to 2014. J Nat Prod 79:629–661. https://doi.org/10.1021/acs.jnatprod.5b01055
Article CAS PubMed Google Scholar
Khalifa SA, Elias N, Farag MA, Chen L, Saeed A, Hegazy MEF et al (2019) Marine natural products: a source of novel anticancer drugs. Mar Drugs 17:491
Article Google Scholar
Bano Mirza S, Bokhari H, Qaiser Fatmi M (2015) Exploring natural products from the biodiversity of Pakistan for computational drug discovery studies: collection, optimization, design and development of a chemical database (ChemDP). https://www.ingentaconnect.com/content/ben/cad/2015/00000011/00000002/art00003 . Accessed 9 Sept 2019
Ahmed J, Preissner S, Dunkel M, Worth CL, Eckert A, Preissner R (2011) SuperSweet—a resource on natural and artificial sweetening agents. Nucleic Acids Res 39:D377–D382. https://doi.org/10.1093/nar/gkq917
Yue Y, Chu G-X, Liu X-S, Tang X, Wang W, Liu G-J et al (2014) TMDB: a literature-curated database for small molecular compounds found from tea. BMC Plant Biol 14:243. https://doi.org/10.1186/s12870-014-0243-1
Article CAS PubMed PubMed Central Google Scholar
Dagan-Wiener A, Di Pizio A, Nissim I, Bahia MS, Dubovski N, Margulis E et al (2019) BitterDB: taste ligands and receptors database in 2019. Nucleic Acids Res 47:D1179–D1185. https://doi.org/10.1093/nar/gky974
Article PubMed Google Scholar
Naveja JJ, Rico-Hidalgo MP, Medina-Franco JL (2018) Analysis of a large food chemical database: chemical space, diversity, and complexity. F1000Research. https://doi.org/10.12688/f1000research.15440.2
Article PubMed PubMed Central Google Scholar
FooDB. http://foodb.ca/ . Accessed 3 Oct 2019
Mahesh SK, Fathima J, Veena VG (2019) Cosmetic potential of natural products: industrial applications. In: Swamy MK, Akhtar MS (eds) Natural Bio-active compounds: volume 2: chemistry, pharmacology and health care practices. Springer Singapore, Singapore, pp 215–250. https://doi.org/10.1007/978-981-13-7205-6_10
Chapter Google Scholar
Dunkel M, Schmidt U, Struck S, Berger L, Gruening B, Hossbach J et al (2009) SuperScent—a database of flavors and scents. Nucleic Acids Res 37:D291–D294. https://doi.org/10.1093/nar/gkn695
Sparks TC, Wessels FJ, Lorsbach BA, Nugent BM, Watson GB (2019) The new age of insecticide discovery-the crop protection industry and the impact of natural products. Pestic Biochem Physiol. https://doi.org/10.1016/j.pestbp.2019.09.002
Füllbeck M, Michalsky E, Dunkel M, Preissner R (2006) Natural products: sources and databases. Nat Prod Rep 23:347–356. https://doi.org/10.1039/B513504B
Yongye AB, Waddell J, Medina-Franco JL (2012) Molecular scaffold analysis of natural products databases in the public domain. Chem Biol Drug Des 80:717–724. https://doi.org/10.1111/cbdd.12011
Johnson SR, Lange BM (2015) Open-access metabolomics databases for natural product research: present capabilities and future potential. Front Bioeng Biotechnol. https://doi.org/10.3389/fbioe.2015.00022
Tawfike AF, Viegelmann C, Edrada-Ebel R (2013) Metabolomics and dereplication strategies in natural products. In: Roessner U, Dias DA (eds) Metabolomics tools for natural product discovery: methods and protocols. Humana Press, Totowa, pp 227–244. https://doi.org/10.1007/978-1-62703-577-4_17
Chen Y, de Bruyn Kops C, Kirchmair J (2017) Data Resources for the computer-guided discovery of bioactive natural products. J Chem Inf Model 57:2099–2111. https://doi.org/10.1021/acs.jcim.7b00341
Pereira F, Aires-de-Sousa J (2018) Computational methodologies in the exploration of marine natural product leads. Mar Drugs 16:236
Harvey AL, Edrada-Ebel R, Quinn RJ (2015) The re-emergence of natural products for drug discovery in the genomics era. Nat Rev Drug Discov. 14:111–129. https://doi.org/10.1038/nrd4510
UniProt Consortium T (2018) UniProt: the universal protein knowledgebase. Nucleic Acids Res 46:2699. https://doi.org/10.1093/nar/gky092
Federhen S (2012) The NCBI Taxonomy database. Nucleic Acids Res 40:D136–D143. https://doi.org/10.1093/nar/gkr1178
omicX. In: omicX [Internet]. https://omictools.com/ . Accessed 9 Oct 2019
Sorokina M (2020) List of natural products databases. Figshare. https://doi.org/10.6084/m9.figshare.11926047.v1
Wilkinson MD, Dumontier M, Aalbersberg IJ, Appleton G, Axton M, Baak A et al (2016) The FAIR Guiding Principles for scientific data management and stewardship. Sci Data. 3:160018. https://doi.org/10.1038/sdata.2016.18
Williams AJ, Martin GE, Rovnyak D (2016) Modern NMR approaches to the structure elucidation of natural products: volume 1: instrumentation and software. Royal Society of Chemistry, London
Google Scholar
Dictionary of Natural Products 28.1. http://dnp.chemnetbase.com/faces/chemical/ChemicalSearch.xhtml;jsessionid=DB01289ACAA79C222859E1CD8A98A894 . Accessed 9 Oct 2019
Reaxys. https://www.reaxys.com/#/search/quick . Accessed 9 Oct 2019
Gabrielson SW (2018) SciFinder. J Med Libr Assoc. 106:588–590. https://doi.org/10.5195/jmla.2018.515
Article PubMed Central Google Scholar
Dictionary of Marine Natural Products 2018. http://dmnp.chemnetbase.com/faces/chemical/ChemicalSearch.xhtml;jsessionid=824F3121F9A123D4684A7A8289F618E2 . Accessed 9 Oct 2019
Dictionary of Food Compounds 2018. http://dfc.chemnetbase.com/faces/chemical/ChemicalSearch.xhtml;jsessionid=60BDE6E1AE536A1C52AFB65A680DC289 . Accessed 18 Oct 2019
Loub WD, Farnsworth NR, Soejarto DD, Quinn ML (1985) NAPRALERT: computer handling of natural product research data. J Chem Inf Model 25:99–103. https://doi.org/10.1021/ci00046a009
Article CAS Google Scholar
Johnson SG (2014) NIST Standard Reference Database 1A v17. In: NIST [Internet]. https://www.nist.gov/srd/nist-standard-reference-database-1a-v17 . Accessed 9 Oct 2019
Blunt JW, Carroll AR, Copp BR, Davis RA, Keyzers RA, Prinsep MR (2018) Marine natural products. Nat Prod Rep 35:8–53. https://doi.org/10.1039/C7NP00052A
MarinLit. http://pubs.rsc.org/marinlit/ . Accessed 9 Oct 2019
Lang G, Mayhudin NA, Mitova MI, Sun L, van der Sar S, Blunt JW, Cole ALJ, Ellis G, Laatsch H, Munro MHG (2008) Evolving trends in the dereplication of natural product extracts: new methodology for rapid, small-scale investigation of natural product extracts. J Nat Prod 71(9):1595–1599. https://doi.org/10.1021/np8002222
Blunt J, Munro M, Upjohn M (2012) The role of databases in marine natural products research. Handb Mar Nat Prod. 1:389–421
AntiBase. https://application.wiley-vch.de/stmdata/antibase.php . Accessed 9 Oct 2019
Wiley-VCH—AntiBase. https://application.wiley-vch.de/stmdata/antibase.php . Accessed 21 Oct 2019
Ikram NKK, Durrant JD, Muchtaridi M, Zalaludin AS, Purwitasari N, Mohamed N et al (2015) A virtual screening approach for identifying plants with anti H5N1 neuraminidase activity. J Chem Inf Model 55:308–316. https://doi.org/10.1021/ci500405g
Ehrman TM, Barlow DJ, Hylands PJ (2010) In silico search for multi-target anti-inflammatories in Chinese herbs and formulas. Bioorg Med Chem 18:2204–2218. https://doi.org/10.1016/j.bmc.2010.01.070
Quinn RJ, Carroll AR, Pham NB, Baron P, Palframan ME, Suraweera L et al (2008) Developing a drug-like natural product library. J Nat Prod 71:464–468. https://doi.org/10.1021/np070526y
Lagunin AA, Druzhilovsky DS, Rudik AV, Filimonov DA, Gawande D, Suresh K et al (2015) Computer evaluation of hidden potential of phytochemicals of medicinal plants of the traditional Indian ayurvedic medicine. Biomeditsinskaia Khimiia. 61:286–297. https://doi.org/10.18097/PBMC20156102286
Berdy J, Kertesz M (1989) Bioactive natural products database: an aid for natural products identification. In: Collier HR (ed) Chemical information. Berlin Heidelberg, Springer, pp 237–251
Hastings J, de Matos P, Dekker A, Ennis M, Harsha B, Kale N et al (2013) The ChEBI reference database and ontology for biologically relevant chemistry: enhancements for 2013. Nucleic Acids Res 41:D456–D463. https://doi.org/10.1093/nar/gks1146
Gaulton A, Hersey A, Nowotka M, Bento AP, Chambers J, Mendez D et al (2017) The ChEMBL database in 2017. Nucleic Acids Res 45:D945–D954. https://doi.org/10.1093/nar/gkw1074
Pence HE, Williams A (2010) ChemSpider: an online chemical information resource. J Chem Educ 87:1123–1124. https://doi.org/10.1021/ed100697w
Hähnke VD, Kim S, Bolton EE (2018) PubChem chemical structure standardization. J. Cheminformatics. 10:36. https://doi.org/10.1186/s13321-018-0293-8
Seiler KP, Kuehn H, Happ MP, DeCaprio D, Clemons PA (2008) Using ChemBank to probe chemical biology. Curr Protoc Bioinforma. 22:14.5.1–14.5.26. https://doi.org/10.1002/0471250953.bi1405s22
Kanehisa M, Furumichi M, Tanabe M, Sato Y, Morishima K (2016) KEGG: new perspectives on genomes, pathways, diseases and drugs. Nucleic Acids Res. https://doi.org/10.1093/nar/gkw1092
Caspi R, Billington R, Fulcher CA, Keseler IM, Kothari A, Krummenacker M et al (2018) The MetaCyc database of metabolic pathways and enzymes. Nucleic Acids Res 46:D633–D639. https://doi.org/10.1093/nar/gkx935
Altman T, Travers M, Kothari A, Caspi R, Karp PD (2013) A systematic comparison of the MetaCyc and KEGG pathway databases. BMC Bioinformatics 14:112
Jeske L, Placzek S, Schomburg I, Chang A, Schomburg D (2019) BRENDA in 2019: a European ELIXIR core data resource. Nucleic Acids Res 47:D542–D549. https://doi.org/10.1093/nar/gky1048
Sitzmann M, Filippov IV, Nicklaus MC (2008) Internet resources integrating many small-molecule databases1. SAR QSAR Environ Res 19:1–9. https://doi.org/10.1080/10629360701843540
King ZA, Lu J, Dräger A, Miller P, Federowicz S, Lerman JA et al (2016) BiGG Models: a platform for integrating, standardizing and sharing genome-scale models. Nucleic Acids Res 44:D515–D522. https://doi.org/10.1093/nar/gkv1049
MassBank of North America (MoNa). http://mona.fiehnlab.ucdavis.edu/ . Accessed 16 Oct 2019
MassBank | European MassBank (NORMAN MassBank) mass spectral database. http://massbank.normandata.eu/MassBank/ . Accessed 16 Oct 2019
MassBank | MSSJ MassBank Mass Spectral DataBase. http://www.massbank.jp/ . Accessed 16 Oct 2019
Guijas C, Montenegro-Burke JR, Domingo-Almenara X, Palermo A, Warth B, Hermann G et al (2018) METLIN: a technology platform for identifying knowns and unknowns. Anal Chem 90:3156–3164. https://doi.org/10.1021/acs.analchem.7b04424
Wishart DS, Feunang YD, Marcu A, Guo AC, Liang K, Vázquez-Fresno R et al (2018) HMDB 4.0: the human metabolome database for 2018. Nucleic Acids Res. 46:D608–D617. https://doi.org/10.1093/nar/gkx1089
Ramirez-Gaona M, Marcu A, Pon A, Guo AC, Sajed T, Wishart NA et al (2017) YMDB 2.0: a significantly expanded version of the yeast metabolome database. Nucleic Acids Res. 45:D440–D445. https://doi.org/10.1093/nar/gkw1058
Wang M, Carver JJ, Phelan VV, Sanchez LM, Garg N, Peng Y et al (2016) Sharing and community curation of mass spectrometry data with Global Natural Products Social Molecular Networking. Nat Biotechnol. 34:828. https://doi.org/10.1038/nbt.3597
Kuhn S, Schlörer NE (2015) Facilitating quality control for spectra assignments of small organic molecules: nmrshiftdb2– a free in-house NMR database with integrated LIMS for academic service laboratories. Magn Reson Chem 53:582–589. https://doi.org/10.1002/mrc.4263
NMRdata. http://www.nmrdata.com/ . Accessed 15 Oct 2019
López-Pérez JL, Therón R, del Olmo E, Díaz D (2007) NAPROC-13: a database for the dereplication of natural product mixtures in bioassay-guided protocols. Bioinformatics 23:3256–3257. https://doi.org/10.1093/bioinformatics/btm516
Fischedick JT, Johnson SR, Ketchum REB, Croteau RB, Lange BM (2015) NMR spectroscopic search module for Spektraris, an online resource for plant natural product identification—Taxane diterpenoids from Taxus × media cell suspension cultures as a case study. Phytochemistry 113:87–95. https://doi.org/10.1016/j.phytochem.2014.11.020
Banerjee P, Erehman J, Gohlke B-O, Wilhelm T, Preissner R, Dunkel M (2015) Super Natural II—a database of natural products. Nucleic Acids Res 43:D935–D939. https://doi.org/10.1093/nar/gku886
Molecular Diversity Preservation International (MDPI). https://www.mdpi.org/ . Accessed 15 Oct 2019
Gu J, Gui Y, Chen L, Yuan G, Lu H-Z, Xu X (2013) Use of natural products as chemical library for drug discovery and network pharmacology. PLoS ONE 8:e62839. https://doi.org/10.1371/journal.pone.0062839
ISDB by oolonek. http://oolonek.github.io/ISDB/ . Accessed 15 Oct 2019
Sterling T, Irwin JJ (2015) ZINC 15—ligand discovery for everyone. J Chem Inf Model 55:2324–2337. https://doi.org/10.1021/acs.jcim.5b00559
Zeng X, Zhang P, He W, Qin C, Chen S, Tao L et al (2018) NPASS: natural product activity and species source database for natural product research, discovery and tool development. Nucleic Acids Res 46:D1217–D1222. https://doi.org/10.1093/nar/gkx1026
Tomiki T, Saito T, Ueki M, Konno H, Asaoka T, Suzuki R et al (2006) RIKEN natural products encyclopedia (RIKEN NPEdia), a chemical database of RIKEN natural products depository (RIKEN NPDepo). J Comput Aid Chem. 7:157–162
Maeda MH, Kondo K (2013) Three-Dimensional Structure Database of Natural Metabolites (3DMET): a novel database of curated 3D structures. J Chem Inf Model 53:527–533. https://doi.org/10.1021/ci300309k
Shen J, Xu X, Cheng F, Liu H, Luo X, Shen J, et al (2003) Virtual screening on natural products for discovering active compounds and target information. https://doi.org/10.2174/0929867033456729 . Accessed 20 May 2019
Blin K, Wolf T, Chevrette MG, Lu X, Schwalen CJ, Kautsar SA et al (2017) antiSMASH 4.0—improvements in chemistry prediction and gene cluster boundary identification. Nucleic Acids Res. 45:W36–W41. https://doi.org/10.1093/nar/gkx319
Skinnider MA, Dejong CA, Rees PN, Johnston CW, Li H, Webster ALH et al (2015) Genomes to natural products PRediction Informatics for Secondary Metabolomes (PRISM). Nucleic Acids Res 43:9645–9662. https://doi.org/10.1093/nar/gkv1012
Crawford MJ, Clardy J (2011) Bacterial symbionts and natural products. Chem Commun. 47:7559–7566. https://doi.org/10.1039/c1cc11574j
Sarethy IP, Srivastava N, Pan S (2019) Endophytes: the unmapped repository for natural products. In: Akhtar MS, Swamy MK, Sinniah UR (eds) Natural Bio-active compounds: volume 1: production and applications. Springer, Singapore, pp 41–70. https://doi.org/10.1007/978-981-13-7154-7_2
Nakamura K, Shimura N, Otabe Y, Hirai-Morita A, Nakamura Y, Ono N et al (2013) KNApSAcK-3D: a three-dimensional structure database of plant metabolites. Plant Cell Physiol 54:e4. https://doi.org/10.1093/pcp/pcs186
Zeng X, Zhang P, Wang Y, Qin C, Chen S, He W et al (2019) CMAUP: a database of collective molecular activities of useful plants. Nucleic Acids Res 47:D1118–D1127. https://doi.org/10.1093/nar/gky965
Miettinen K, Iñigo S, Kreft L, Pollier J, De Bo C, Botzki A et al (2018) The TriForC database: a comprehensive up-to-date resource of plant triterpene biosynthesis. Nucleic Acids Res 46:D586–D594. https://doi.org/10.1093/nar/gkx925
Boonen J, Bronselaer A, Nielandt J, Veryser L, De Tré G, De Spiegeleer B (2012) Alkamid database: chemistry, occurrence and functionality of plant N -alkylamides. J Ethnopharmacol 142:563–590. https://doi.org/10.1016/j.jep.2012.05.038
Klementz D, Döring K, Lucas X, Telukunta KK, Erxleben A, Deubel D et al (2016) StreptomeDB 2.0—an extended resource of natural products produced by streptomycetes. Nucleic Acids Res. 44:D509–D514. https://doi.org/10.1093/nar/gkv1319
Natural Products Atlas. https://www.npatlas.org/joomla/ . Accessed 16 Oct 2019
Nupur LNU, Vats A, Dhanda SK, Raghava GPS, Pinnaka AK, Kumar A (2016) ProCarDB: a database of bacterial carotenoids. BMC Microbiol 16:96. https://doi.org/10.1186/s12866-016-0715-6
Huang W, Brewer LK, Jones JW, Nguyen AT, Marcu A, Wishart DS et al (2018) PAMDB: a comprehensive Pseudomonas aeruginosa metabolome database. Nucleic Acids Res 46:D575–D580. https://doi.org/10.1093/nar/gkx1061
Lichen Database. In: MTBLS999: A database of high-resolution MS/MS spectra for lichen metabolites [Internet]. https://www.ebi.ac.uk/metabolights/MTBLS999 . Accessed 16 Oct 2019
Haug K, Salek RM, Conesa P, Hastings J, de Matos P, Rijnbeek M et al (2013) MetaboLights—an open-access general-purpose repository for metabolomics studies and associated meta-data. Nucleic Acids Res 41:D781–D786. https://doi.org/10.1093/nar/gks1004
Organization WH (1999) WHO monographs on selected medicinal plants, vol 2. World Health Organization, Geneva
World Health Organization (2009) WHO monographs on selected medicinal plants, vol 4. World Health Organization, Geneva
Polur H, Joshi T, Workman CT, Lavekar G, Kouskoumvekaki I (2011) Back to the roots: prediction of biologically active natural products from ayurveda traditional medicine. Mol Inform. 30:181–187. https://doi.org/10.1002/minf.201000163
Palhares RM, Gonçalves Drummond M, dos Santos Alves Figueiredo Brasil B, Pereira Cosenza G, das Graças Lins Brandão M, Oliveira G (2015) Medicinal plants recommended by the World Health Organization: DNA barcode identification associated with chemical analyses guarantees their quality. PLoS ONE. https://doi.org/10.1371/journal.pone.0127866
Xu J, Yang Y (2009) Traditional Chinese medicine in the Chinese health care system. Health Policy 90:133–139. https://doi.org/10.1016/j.healthpol.2008.09.003
Yuan H, Ma Q, Ye L, Piao G (2016) The traditional medicine and modern medicine from natural products. Molecules 21:559. https://doi.org/10.3390/molecules21050559
Article CAS PubMed Central Google Scholar
Chen CYC (2011) TCM Database@Taiwan: the World’s Largest Traditional Chinese Medicine database for drug screening in silico. PLOS ONE. 6:e15939. https://doi.org/10.1371/journal.pone.0015939
Chang K-W, Tsai T-Y, Chen K-C, Yang S-C, Huang H-J, Chang T-T et al (2011) iSMART: an integrated cloud computing web server for traditional Chinese medicine for online virtual screening, de novo evolution and drug design. J Biomol Struct Dyn 29:243–250. https://doi.org/10.1080/073911011010524988
Huang J, Zheng Y, Wu W, Xie T, Yao H, Pang X et al (2015) CEMTDD: the database for elucidating the relationships among herbs, compounds, targets and related diseases for Chinese ethnic minority traditional drugs. Oncotarget. 6:17675–17684. https://doi.org/10.18632/oncotarget.3789
Qiao X, Hou T, Zhang W, Guo S, Xu X (2002) A 3D structure database of components from Chinese traditional medicinal herbs. J Chem Inf Comput Sci 42:481–489. https://doi.org/10.1021/ci010113h
Fang X, Shao L, Zhang H, Wang S (2005) CHMIS-C: a comprehensive herbal medicine information system for cancer. J Med Chem 48:1481–1488. https://doi.org/10.1021/jm049838d
Xu H-Y, Zhang Y-Q, Liu Z-M, Chen T, Lv C-Y, Tang S-H et al (2019) ETCM: an encyclopaedia of traditional Chinese medicine. Nucleic Acids Res 47:D976–D982. https://doi.org/10.1093/nar/gky987
Kim S-K, Nam S, Jang H, Kim A, Lee J-J (2015) TM-MC: a database of medicinal materials and chemical compounds in Northeast Asian traditional medicine. BMC Complement Altern Med. 15:218. https://doi.org/10.1186/s12906-015-0758-5
TCMID: traditional Chinese medicine integrative database for herb molecular mechanism analysis. https://www.ncbi.nlm.nih.gov/pmc/articles/PMC3531123/ . Accessed 29 Apr 2019
Ru J, Li P, Wang J, Zhou W, Li B, Huang C et al (2014) TCMSP: a database of systems pharmacology for drug discovery from herbal medicines. J Cheminformatics. 6:13. https://doi.org/10.1186/1758-2946-6-13
Li B, Ma C, Zhao X, Hu Z, Du T, Xu X et al (2018) YaTCM: yet another traditional Chinese medicine database for drug discovery. Comput Struct Biotechnol J. 16:600–610. https://doi.org/10.1016/j.csbj.2018.11.002
Mohanraj K, Karthikeyan BS, Vivek-Ananth RP, Chand RPB, Aparna SR, Mangalapandi P et al (2018) IMPPAT: a curated database of Indian medicinal plants, phytochemistry and therapeutics. Sci Rep. https://doi.org/10.1038/s41598-018-22631-z
Potshangbam AM, Polavarapu R, Rathore RS, Naresh D, Prabhu NP, Potshangbam N et al (2019) MedPServer: a database for identification of therapeutic targets and novel leads pertaining to natural products. Chem Biol Drug Des 93:438–446. https://doi.org/10.1111/cbdd.13430
Meetei PA, Singh P, Nongdam P, Prabhu NP, Rathore R, Vindal V (2012) NeMedPlant: a database of therapeutic applications and chemical constituents of medicinal plants from north-east region of India. Bioinformation. 8:209–211. https://doi.org/10.6026/97320630008209
Pathania S, Ramakrishnan SM, Bagler G (2015) Phytochemica: a platform to explore phytochemicals of medicinal plants. Database. https://doi.org/10.1093/database/bav075
Yanuar A, Mun’im A, Lagho ABA, Syahdi RR, Rahmat M, Suhartanto H (2011) Medicinal plants database and three dimensional structure of the chemical compounds from medicinal plants in Indonesia. ArXiv11117183 Q-Bio. http://arxiv.org/abs/1111.7183 . Accessed 22 Oct 2019
Tung C-W, Lin Y-C, Chang H-S, Wang C-C, Chen I-S, Jheng J-L et al (2014) TIPdb-3D: the three-dimensional structure database of phytochemicals from Taiwan indigenous plants. Database. https://doi.org/10.1093/database/bau055
Ntie-Kang F, Zofou D, Babiaka SB, Meudom R, Scharfe M, Lifongo LL et al (2013) AfroDb: a select highly potent and diverse natural product library from African medicinal plants. PLoS ONE 8:e78085. https://doi.org/10.1371/journal.pone.0078085
Ntie-Kang F, Onguéné PA, Fotso GW, Andrae-Marobela K, Bezabih M, Ndom JC et al (2014) Virtualizing the p-ANAPL library: a step towards drug discovery from African medicinal plants. PLoS ONE 9:e90655. https://doi.org/10.1371/journal.pone.0090655
Ntie-Kang F, Nwodo JN, Ibezim A, Simoben CV, Karaman B, Ngwa VF et al (2014) Molecular modeling of potential anticancer agents from African medicinal plants. J Chem Inf Model 54:2433–2450. https://doi.org/10.1021/ci5003697
Onguéné PA, Ntie-Kang F, Mbah JA, Lifongo LL, Ndom JC, Sippl W et al (2014) The potential of anti-malarial compounds derived from African medicinal plants, part III: an in silico evaluation of drug metabolism and pharmacokinetics profiling. Org Med Chem Lett. 4:6. https://doi.org/10.1186/s13588-014-0006-x
Ibezim A, Debnath B, Ntie-Kang F, Mbah CJ, Nwodo NJ (2017) Binding of anti-Trypanosoma natural products from African flora against selected drug targets: a docking study. Med Chem Res 26:562–579. https://doi.org/10.1007/s00044-016-1764-y
Ntie-Kang F, Mbah JA, Mbaze LM, Lifongo LL, Scharfe M, Hanna JN et al (2013) CamMedNP: building the Cameroonian 3D structural natural products database for virtual screening. BMC Complement Altern Med. 13:88. https://doi.org/10.1186/1472-6882-13-88
Ntie-Kang F, Amoa Onguéné P, Scharfe M, Owono LCO, Megnassan E, Meva’a Mbaze L et al (2014) ConMedNP: a natural product library from Central African medicinal plants for drug discovery. RSC Adv. 4:409–419. https://doi.org/10.1039/c3ra43754j
Bultum LE, Woyessa AM, Lee D (2019) ETM-DB: integrated Ethiopian traditional herbal medicine and phytochemicals database. BMC Complement Altern Med. 19:212. https://doi.org/10.1186/s12906-019-2634-1
Wishart DS, Feunang YD, Guo AC, Lo EJ, Marcu A, Grant JR et al (2018) DrugBank 5.0: a major update to the DrugBank database for 2018. Nucleic Acids Res. 46:D1074–D1082. https://doi.org/10.1093/nar/gkx1037
DrugBank | nutraceutical search. https://www.drugbank.ca/drugs?utf8=%E2%9C%93&nutraceutical=1&filter=true . Accessed 17 Oct 2019
Law V, Knox C, Djoumbou Y, Jewison T, Guo AC, Liu Y et al (2014) DrugBank 4.0: shedding new light on drug metabolism. Nucleic Acids Res. 42:D1091–D1097. https://doi.org/10.1093/nar/gkt1068
Gilson MK, Liu T, Baitaluk M, Nicola G, Hwang L, Chong J (2016) BindingDB in 2015: a public database for medicinal chemistry, computational chemistry and systems pharmacology. Nucleic Acids Res 44:D1045–D1053. https://doi.org/10.1093/nar/gkv1072
Novel Antibiotics Database. http://www.antibiotics.or.jp/journal/database/database-top.htm . Accessed 18 Oct 2019
Tomasulo P (2002) ChemIDplus-super source for chemical and drug information. Med Ref Serv Q. 21:53–59. https://doi.org/10.1300/J115v21n01_04
Ye H, Ye L, Kang H, Zhang D, Tao L, Tang K et al (2011) HIT: linking herbal active ingredients to targets. Nucleic Acids Res 39:D1055–D1059. https://doi.org/10.1093/nar/gkq1165
Kang H, Tang K, Liu Q, Sun Y, Huang Q, Zhu R et al (2013) HIM-herbal ingredients in vivo metabolism database. J Cheminformatics. 5:28. https://doi.org/10.1186/1758-2946-5-28
Choi H, Cho SY, Pak HJ, Kim Y, Choi J, Lee YJ et al (2017) NPCARE: database of natural products and fractional extracts for cancer regulation. J Cheminformatics. 9:2. https://doi.org/10.1186/s13321-016-0188-5
Vetrivel U, Subramanian N, Pilla K (2009) InPACdb—Indian plant anticancer compounds database. Bioinformation 4:71–74
Dr.V,Umashankar (2018) InPACdb | Indian-Plant-Anticancer-Compound-DB. https://github.com/inpacdb/Indian-Plant-Anticancer-Compound-DB-inpacdb . Accessed 17 Oct 2019
Mangal M, Sagar P, Singh H, Raghava GPS, Agarwal SM (2013) NPACT: naturally occurring plant-based anti-cancer compound-activity-target database. Nucleic Acids Res 41:D1124–D1129. https://doi.org/10.1093/nar/gks1047
Compound Sets—NCI DTP Data—National Cancer Institute—Confluence Wiki. https://wiki.nci.nih.gov/display/NCIDTPdata/Compound+Sets . Accessed 18 Oct 2019
Zhang R, Lin J, Zou Y, Zhang X-J, Xiao W-L (2019) Chemical space and biological target network of anti-inflammatory natural products. J Chem Inf Model 59:66–73. https://doi.org/10.1021/acs.jcim.8b00560
Sharma A, Dutta P, Sharma M, Rajput NK, Dodiya B, Georrge JJ et al (2014) BioPhytMol: a drug discovery community resource on anti-mycobacterial phytomolecules and plant extracts. J Cheminformatics. 6:46. https://doi.org/10.1186/s13321-014-0046-2
OSM—Open Source Malaria. http://opensourcemalaria.org/ . Accessed 18 Oct 2019
Williamson AE, Ylioja PM, Robertson MN, Antonova-Koch Y, Avery V, Baell JB et al (2016) Open Source Drug Discovery: highly potent antimalarial compounds derived from the Tres Cantos Arylpyrroles. ACS Cent Sci. 2:687–701. https://doi.org/10.1021/acscentsci.6b00086
Rothwell JA, Perez-Jimenez J, Neveu V, Medina-Remón A, M’Hiri N, García-Lobato P et al (2013) Phenol-Explorer 3.0: a major update of the Phenol-Explorer database to incorporate data on the effects of food processing on polyphenol content. Database. https://doi.org/10.1093/database/bat070
PhytoHub. http://phytohub.eu/ . Accessed 16 Oct 2019
Neveu V, Moussy A, Rouaix H, Wedekind R, Pon A, Knox C et al (2017) Exposome-Explorer: a manually-curated database on biomarkers of exposure to dietary and environmental factors. Nucleic Acids Res 45:D979–D984. https://doi.org/10.1093/nar/gkw980
Lim E, Pon A, Djoumbou Y, Knox C, Shrivastava S, Guo AC et al (2010) T3DB: a comprehensively annotated database of common toxins and their targets. Nucleic Acids Res 38:D781–D786. https://doi.org/10.1093/nar/gkp934
He Q-Y, He Q-Z, Deng X-C, Yao L, Meng E, Liu Z-H et al (2008) ATDB: a uni-database platform for animal toxins. Nucleic Acids Res 36:D293–D297. https://doi.org/10.1093/nar/gkm832
International Venom and Toxin Database. http://www.kingsnake.com/toxinology/
Snake Neurotoxin Database. http://sdmc.i2r.a-star.edu.sg/Templar/DB/snake_neurotoxin/
MOLLUSK toxin database. http://research.i2r.a-star.edu.sg/MOLLUSK/
Srinivasan KN, Gopalakrishnakone P, Tan PT, Chew KC, Cheng B, Kini RM et al (2002) SCORPION, a molecular database of scorpion toxins. Toxicon 40:23–31. https://doi.org/10.1016/S0041-0101(01)00182-9
Günthardt BF, Hollender J, Hungerbühler K, Scheringer M, Bucheli TD (2018) Comprehensive toxic plants-phytotoxins database and its application in assessing aquatic micropollution potential. J Agric Food Chem 66:7577–7588. https://doi.org/10.1021/acs.jafc.8b01639
Yabuzaki J (2017) Carotenoids Database: structures, chemical fingerprints and distribution among organisms. Database J Biol Databases Curation. https://doi.org/10.1093/database/bax004
Rodriguez-Amaya DB, Kimura M, Godoy HT, Amaya-Farfan J (2008) Updated Brazilian database on food carotenoids: factors affecting carotenoid composition. J Food Compos Anal 21:445–463. https://doi.org/10.1016/j.jfca.2008.04.001
Pilón-Jiménez BA, Saldívar-González FI, Díaz-Eufracio BI, Medina-Franco JL (2019) BIOFACQUIM: a Mexican compound database of natural products. Biomolecules. 9:31. https://doi.org/10.3390/biom9010031
Pilon AC, Valli M, Dametto AC, Pinto MEF, Freire RT, Castro-Gamboa I et al (2017) NuBBEDB: an updated database to uncover chemical and biological information from Brazilian biodiversity. Sci Rep. 7:7215. https://doi.org/10.1038/s41598-017-07451-x
UEFS Natural Products. http://zinc12.docking.org/catalogs/uefsnp . Accessed 6 Nov 2019
Ntie-Kang F, Telukunta KK, Döring K, Simoben CV, Moumbock AF, Malange YI et al (2017) NANPDB: a resource for natural products from Northern African sources. J Nat Prod. 80:2067–2076. https://doi.org/10.1021/acs.jnatprod.7b00283
Hatherley R, Brown DK, Musyoka TM, Penkler DL, Faya N, Lobb KA et al (2015) SANCDB: a South African natural compound database. J Cheminformatics 7:29. https://doi.org/10.1186/s13321-015-0080-8
Derese S, Oyim J, Rogo M, Ndakala A (2015) Mitishamba database: a web based in silico database of natural products from Kenya plants. University of Nairobi, Nairobi
Ashfaq UA, Mumtaz A, ul-Qamar T, Fatima T (2013) MAPS database: medicinal plant activities, phytochemical and structural database. Bioinformation 9:993–995. https://doi.org/10.6026/97320630009993
Nguyen-Vo T-H, Le T, Pham D, Nguyen T, Le P, Nguyen A et al (2019) VIETHERB: a database for Vietnamese herbal species. J Chem Inf Model 59:1–9. https://doi.org/10.1021/acs.jcim.8b00399
Journal of Natural Products. https://pubs.acs.org/journal/jnprdf
Marine Drugs. https://www.mdpi.com/journal/marinedrugs
A database of natural products and chemical entities from marine habitat. http://www.bioinformation.net/003/003000032008.htm . Accessed 6 Nov 2019
Lei J, Zhou J (2002) A marine natural product database. J Chem Inf Comput Sci 42:742–748. https://doi.org/10.1021/ci010111x
Sagar S, Kaur M, Radovanovic A, Bajic VB (2013) Dragon exploration system on marine sponge compounds interactions. J Cheminformatics 5:11. https://doi.org/10.1186/1758-2946-5-11
Davis GDJ, Vasanthi AHR (2011) Seaweed metabolite database (SWMD): a database of natural compounds from marine algae. Bioinformation 5:361–364
Ambinter-Greenpharma natural compound library (GPNCL). In: Greenpharma [Internet]. https://www.greenpharma.com/products/compound-librairies/ . Accessed 9 Oct 2019
ChemBridge | Screening Library | Diversity Libraries. https://www.chembridge.com/screening_libraries/diversity_libraries/ . Accessed 16 Oct 2019
LOPAC1280. Library of pharmacologically active compounds. In: Sigma-Aldrich [Internet]. https://www.sigmaaldrich.com/life-science/cell-biology/bioactive-small-molecules/lopac1280-navigator.html . Accessed 16 Oct 2019
Prestwick Chemical. The Prestwick Phytochemical Library, a collection of natural products. http://www.prestwickchemical.com/libraries-screening-lib-phyto.html . Accessed 16 Oct 2019
Targetmol | Natural Compound Library. https://www.targetmol.com/compound-library/Natural-Compounds-Library . Accessed 16 Oct 2019
AnalytiCon Discovery, Screening Libraries. In: AnalytiCon Discovery [Internet]. https://ac-discovery.com/screening-libraries/ . Accessed 16 Oct 2019
InterBioScreen | Natural Compounds. https://www.ibscreen.com/natural-compounds . Accessed 9 Oct 2019
INDOFINE Chemical Company. http://www.indofinechemical.com/Media/sdf/sdf_files.aspx . Accessed 16 Oct 2019
Pi Chemicals System. http://www.pipharm.com/catalog_products/list?category=28 . Accessed 16 Oct 2019
Specs. Compound management services and research compounds for the life science industry. https://www.specs.net/index.php . Accessed 16 Oct 2019
ZINC Specs Natural Products. http://zinc.docking.org/catalogs/specsnp/ . Accessed 16 Oct 2019
Ertl P, Roggo S, Schuffenhauer A (2008) Natural product-likeness score and its application for prioritization of compound libraries. J Chem Inf Model 48:68–74. https://doi.org/10.1021/ci700286x
Sorokina M, Steinbeck C (2019) NaPLeS: a natural products likeness scorer—web application and database. J Cheminformatics. 11:55. https://doi.org/10.1186/s13321-019-0378-z
Willighagen EL, Mayfield JW, Alvarsson J, Berg A, Carlsson L, Jeliazkova N et al (2017) The Chemistry Development Kit (CDK) v2.0: atom typing, depiction, molecular formulas, and substructure searching. J Cheminformatics 9:33. https://doi.org/10.1186/s13321-017-0220-4
Otasek D, Morris JH, Bouças J, Pico AR, Demchak B (2019) Cytoscape Automation: empowering workflow-based network analysis. Genome Biol 20:185. https://doi.org/10.1186/s13059-019-1758-4
Download references
Acknowledgements
Not applicable.
This work was supported by the German Research Foundation within the framework CRC1127 ChemBioSys.
Author information
Authors and affiliations.
University Friedrich-Schiller, Lessing Strasse 8, 07743, Jena, Germany
Maria Sorokina & Christoph Steinbeck
You can also search for this author in PubMed Google Scholar
Contributions
MS researched the mentioned natural products resources, compiled the COCONUT dataset from them and designed the web application. CS conceived and oversaw the project. Both authors read and approved this manuscript.
Corresponding author
Correspondence to Maria Sorokina .
Ethics declarations
Competing interests.
The authors declare that they have no competing interests.
Additional information
Publisher's note.
Springer Nature remains neutral with regard to jurisdictional claims in published maps and institutional affiliations.
Supplementary information
Additional file 1..
Overlap (in percent) of compound content between open natural products databases.
Rights and permissions
Open Access This article is licensed under a Creative Commons Attribution 4.0 International License, which permits use, sharing, adaptation, distribution and reproduction in any medium or format, as long as you give appropriate credit to the original author(s) and the source, provide a link to the Creative Commons licence, and indicate if changes were made. The images or other third party material in this article are included in the article's Creative Commons licence, unless indicated otherwise in a credit line to the material. If material is not included in the article's Creative Commons licence and your intended use is not permitted by statutory regulation or exceeds the permitted use, you will need to obtain permission directly from the copyright holder. To view a copy of this licence, visit http://creativecommons.org/licenses/by/4.0/ . The Creative Commons Public Domain Dedication waiver ( http://creativecommons.org/publicdomain/zero/1.0/ ) applies to the data made available in this article, unless otherwise stated in a credit line to the data.
Reprints and permissions
About this article
Cite this article.
Sorokina, M., Steinbeck, C. Review on natural products databases: where to find data in 2020. J Cheminform 12 , 20 (2020). https://doi.org/10.1186/s13321-020-00424-9
Download citation
Received : 04 February 2020
Accepted : 22 March 2020
Published : 03 April 2020
DOI : https://doi.org/10.1186/s13321-020-00424-9
Share this article
Anyone you share the following link with will be able to read this content:
Sorry, a shareable link is not currently available for this article.
Provided by the Springer Nature SharedIt content-sharing initiative
- Natural products
- Drug discovery
Journal of Cheminformatics
ISSN: 1758-2946
- Submission enquiries: [email protected]
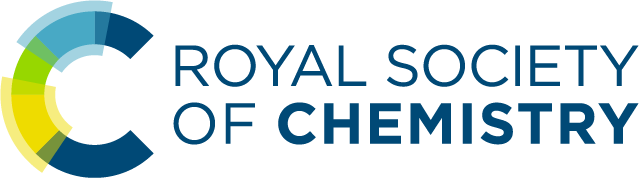
Journals, books & databases
- Our journals
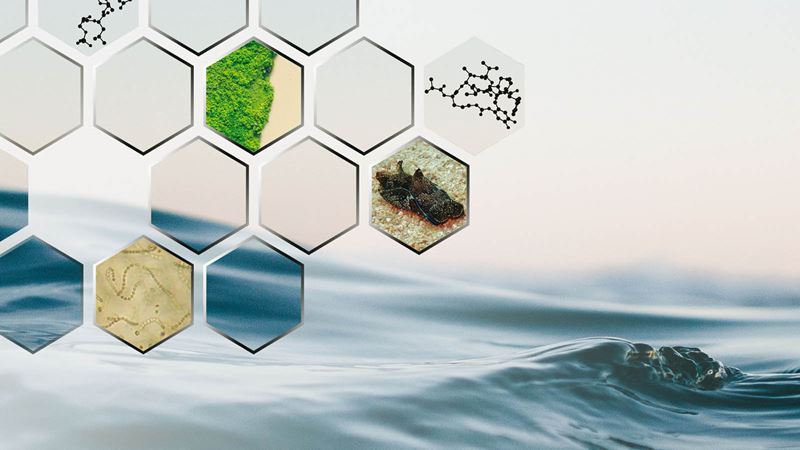
Natural Product Reports
The journal for high impact, critical reviews in natural products research and related areas
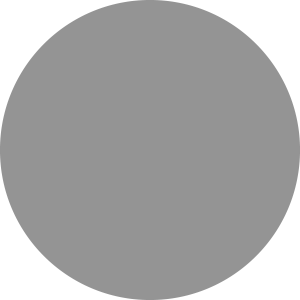
You can find details about how to access information remotely in this step-by-step guide . The guide will also help if for any reason you have difficulty accessing the content you want.
What would you like to know about this journal?
Natural Product Reports is a Transformative Journal and Plan S compliant
Impact factor: 11.9*
Time to first decision (all decisions): 42.0 days**
Time to first decision (peer reviewed only): 42.5 days***
Chair: Tobias Gulder
Indexed in MEDLINE
CiteScore: 21.1****
Open access publishing options available
Read this journal
Submit a synopsis
Sign up for regular email alerts
View all journal metrics
Publish open access
Meet the team
Journal scope.
Natural Product Reports ( NPR) is a critical review journal that stimulates progress in all areas of natural products research, including isolation, structural and stereochemical determination, biosynthesis, biological activity and synthesis.
The scope of the journal is very broad, and many reviews discuss the role of natural products in the wider bioinorganic, bioorganic and chemical biology communities. Areas covered include the following:
- Nucleic acids
- Chemical ecology
- Carbohydrates
- Primary and secondary metabolism
- Analytical techniques
NPR articles are designed to give an interesting insight into the topic, focussing on the key developments that have shaped a field rather than giving a very comprehensive overview of all results.
Authors are encouraged to include their own perspective on developments, trends and future directions.
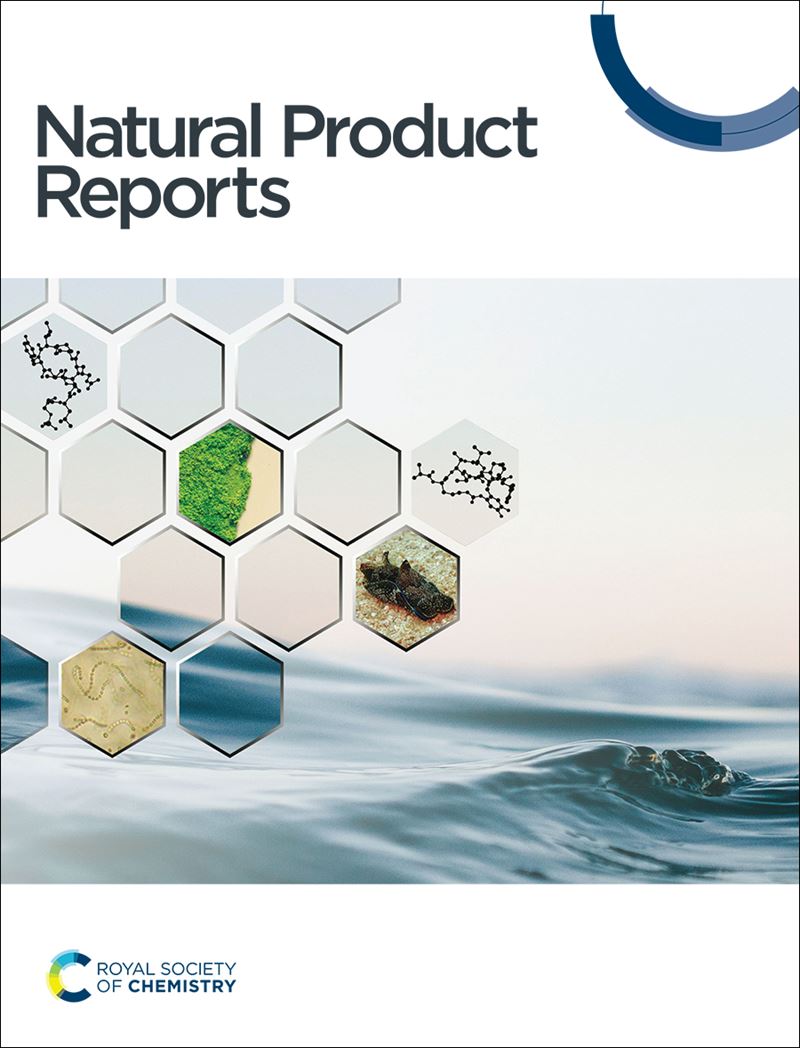
Natural Product Reports Lectureship award
This Lectureship recognises an outstanding emerging investigator who has made a significant contribution to a natural products-related area of the chemical sciences, providing a platform to showcase their research to the wider scientific community. The NPR Lectureship is awarded annually through a process whereby nominations of candidates are invited from our community.
You can read about eligibility, how to nominate, see deadlines and all of the award winners.
Find out who is on the editorial and advisory boards for the Natural Product Reports journal.
Tobias Gulder , Technical University of Dresden and Helmholtz Institute for Pharmaceutical Research Saarland, Germany
Editorial board members
Heike Brötz-Oesterhelt , University of Tübingen, Germany
Nadja Cech , University of North Carolina, Greensboro, USA
Alessandra Eustaquio , University of Illinois at Chicago, USA
Roger Linington , Simon Fraser University, Canada
Dong-Chan Oh , Seoul National University, South Korea
Cassandra Quave , Emory University, USA
Margherita Sosio , Naicons Srl, Milan, Italy
Eriko Takano , University of Manchester, UK
Hidetoshi Tokuyama , Tohoku University, Japan
Christopher Vanderwal , University of California, Irvine, USA
Changsheng Zhang , South China Sea Institute of Oceanology, China Academy of Sciences, China
Giovanni Appendino , Universita del Piemonte Orientale, Italy
Roberto Berlinck , University of Sao Paulo, Brazil
Carole Bewley , National Institutes of Health, USA
Christopher Boddy , University of Ottawa, Canada
Robert Britton , Simon Fraser University, Canada
Margaret A Brimble , University of Auckland, New Zealand
Mark Brönstrup , Helmholtz Centre for Infection Research, Germany
Guy Carter , Carter-Bernan Consulting, USA
Russell Cox , Leibniz Universität Hannover, Germany
Pieter Dorrestein , University of California, San Diego, USA
Katherine Duncan , University of Strathclyde, UK
Neha Garg , Georgia Institute of Technology, USA
Susana P. Gaudêncio , Nova University Lisbon, Portugal
Olga Genilloud , Fundación MEDINA, Spain
Rebecca Goss , St. Andrews University, UK
Seth Herzon , Yale University, USA
Chambers Hughes , University of Tuebingen, Germany
Marcel Jaspars , University of Aberdeen, UK
Martin Kaltenpoth , Johannes Gutenberg University Mainz, Germany
Andreas Kirschning , University of Hannover, Germany
Julia Kubanek , Georgia Institute of Technology, USA
Wen Liu , Shanghai Institute of Organic Chemistry, China
Sandra Loesgen , University of Florida, USA
Hendrik Luesch , University of Florida, USA
Dawei Ma , Shanghai Institute of Organic Chemistry, China
Marnix Medema , Wageningen University, Netherlands
Fidele Ntie-Kang , University of Buea, Cameroon
Sarah O'Connor , Max Planck Institute for Chemical Ecology, Jena, Germany
Jörn Piel , ETH Zurich, Switzerland
Jürgen Rohr , University of Kentucky, USA
Martin Schmeing , McGill University, Canada
Stefan Schulz , TU Braunschweig, Germany
Michael Sherburn , Australia National University, Australia
Thomas J Simpson , University of Bristol, UK
Janet Smith , University of Michigan, USA
Renxiang Tan , Nanjing University, China
Dirk Trauner , Ludwig-Maximilian University Munich, Germany
Reiko Ueoka , Kitasato Universit, Japan
Kira Weissman , Lorraine University, France
Craig Williams , The University of Queensland, Australia
Zhen Yang , Peking University, China
Yeo Joon Yoon , Seoul National University, Korea
Weidong Zhang , Second Military Medical University, Shanghai, China
Katie Lim , Executive Editor
Jack Washington , Deputy Editor
Daniel Robertshaw , Development Editor
Sarah Anthony , Editorial Production Manager
Nicola Burton , Publishing Editor
Tom Cozens , Publishing Editor
Ryan Kean , Publishing Editor
Roxane Owen , Publishing Editor, ORCID 0000-0002-4553-233X
Lauren Yarrow-Wright , Publishing Editor
Andrea Whiteside , Publishing Assistant
Sam Keltie , Publisher, Journals, ORCID 0000-0002-9369-8414
Article types
Natural Product Reports publishes:
Hot off the press articles
Reviews, Highlights and Viewpoints in NPR are generally solicited by members of the Editorial board; however we are happy to consider submission enquiries from authors. If you are interested in writing an article for NPR please contact the Editorial office with a brief synopsis.
Synopses for all proposed articles are considered by the board before a decision on the commissioning of the full article is taken.
Submitting a synopsis to NPR
Review articles
Critical reviews of key areas of research, focusing on key developments rather than comprehensive accounts of everything that has occurred in the field; generally 10–25 pages in length.
Highlight articles
Concise, non-comprehensive reviews which give a 'snapshot' of a hot or specialist research area; 4–8 pages in length, generally with up to 8 display items and 50 references.
Viewpoint articles
Short articles that focus on some of the key challenges, issues or developments in natural products research. They can be "opinion" style articles, which give the author's perspective on a particular issue, backed up by the literature. Up to 4 pages in length, generally with up to 3 display items and 20 references.
Bi-monthly digests of the latest natural products research, written by Bob Hill and Andrew Sutherland at the University of Glasgow, UK.
Transparent peer review
As part of our commitment to transparency and open science, Natural Product Reports is now offering authors the option of transparent peer review, where the editor’s decision letter, reviewers’ comments and authors’ response for all versions of the manuscript will be published alongside the article under an Open Access Creative Commons licence (CC-BY) . Please note comments and decisions on synopses will not be published.
Reviewers will remain anonymous unless they choose to sign their report.
Find out more about our transparent peer review policy
Journal specific guidelines
Guidelines on writing titles, abstracts & table of contents entry.
The title, abstract and table of contents entry (graphical abstract) are the first parts of your manuscript that editors, referees and potential readers will see, and once published they play a major part in a researcher’s decision to read your article. Therefore it’s important that these clearly and concisely summarise the topic of your review and its significance.
The title should be short and straightforward to appeal to a general reader, but detailed enough to properly reflect the contents of your review article.
- Keep it relatively short – between 8 and 15 words is ideal.
- Use easily recognisable words and phrases that can be read quickly.
- Use general or well-known terms for compounds and procedures rather than very specialised terms or nomenclature.
- Avoid using non-standard abbreviations and symbols.
- Use keywords and familiar, searchable terms – these can increase the chances of your article appearing in search results. Around 70% of our readers come directly via search engines.
The abstract is a single paragraph which summarises the contents of your article. It will help readers to decide whether your article is of interest to them.
- The length can vary from 50 to 250 words (or up to 100 words for Highlight articles), but it should always be concise and easy to read with recognisable words and phrases.
- It should summarise the research area that your review covers, including why it is important.
- Like your title, make sure you use familiar, searchable terms and keywords.
Table of contents entry
A table of contents entry (graphical abstract) is required, which should be submitted at the revision stage. This should include an eye-catching graphic and 1-2 sentence(s) of text to summarise the key findings of the article to the reader. It will appear in the table of contents and feeds – for example, RSS feeds.
The graphic should:
- Be simple, but informative.
- Capture the reader’s attention (the use of colour is encouraged).
- Include a structure, scheme, graph, drawing, photograph or combination that conveys the message of the article. Please note, complex schematics or spectra should be avoided.
- Be original, unpublished artwork created by one of the co-authors. Preferably, the graphic should not be reused and appear again within the article.
- Be suitable for, and uphold the standards of, a scholarly publication that has a global reach.
- Not contain any elements that are offensive or inappropriate, in particular words or images that are discriminatory.
- Not contain large amounts of text. Text should be limited to the labelling of compounds, reaction arrows and diagrams, with long phrases or sentences being avoided. Any text should be clearly legible to a reader.
- Not contain logos, trademarks or brands names.
The text should:
- Be concise and focus only on the key findings of the manuscript and their importance, not the processes used; think about what would grab the attention of the potential reader and would encourage them to read the full article.
- Avoid repeating or paraphrasing the title or abstract.
Table of contents specifications:
- The figure should be a maximum size of 8 cm wide x 4 cm high.
- Figures should be supplied as TIFF files, with a resolution of 600 dpi or greater.
- The text supplied should be 1-2 sentences long, using a maximum of 250 characters.
Chemical proteomics approaches for identifying the cellular targets of natural products From DOI: 10.1039/C6NP00001K
Dereplication, sequencing and identification of peptidic natural products: from genome mining to peptidogenomics to spectral networks From DOI: 10.1039/C5NP00050E
A twist of nature – the significance of atropisomers in biological systems From DOI: 10.1039/C4NP00121D
This highlight provides an overview of recent progress towards elucidating the structure, biosynthesis, and mode of action of colibactin, a genotoxic secondary metabolite synthesized by human gut bacteria. While isolating colibactin has been problematic, efforts to characterize its biosynthesis have provided critical information that has led to a rapid increase in our knowledge of this metabolite's structure and function. Major questions and gaps remain however, and the broader lessons learned from studying colibactin underscore central challenges to be faced in the genomics era of natural product research and in efforts to understand the human microbiome. From DOI: 10.1039/C5NP00091B Deconvoluting the mode of action of natural products and drugs remains one of the biggest challenges in chemistry and biology today. Chemical proteomics is a growing area of chemical biology that seeks to design small molecule probes to understand protein function. In the context of natural products, chemical proteomics can be used to identify the protein binding partners or targets of small molecules in live cells. Here, we highlight recent examples of chemical probes based on natural products and their application for target identification. The review focuses on probes that can be covalently linked to their target proteins (either via intrinsic chemical reactivity or via the introduction of photocrosslinkers), and can be applied “in situ” – in living systems rather than cell lysates. We also focus here on strategies that employ a click reaction, the copper-catalysed azide–alkyne cycloaddition reaction (CuAAC), to allow minimal functionalisation of natural product scaffolds with an alkyne or azide tag. We also discuss ‘competitive mode’ approaches that screen for natural products that compete with a well-characterised chemical probe for binding to a particular set of protein targets. Fuelled by advances in mass spectrometry instrumentation and bioinformatics, many modern strategies are now embracing quantitative proteomics to help define the true interacting partners of probes, and we highlight the opportunities this rapidly evolving technology provides in chemical proteomics. Finally, some of the limitations and hallenges of chemical proteomics approaches are discussed. From DOI: 10.1039/C6NP00001K
While recent breakthroughs in the discovery of peptide antibiotics and other Peptidic Natural Products (PNPs) raise a challenge for developing new algorithms for their analyses, the computational technologies for high-throughput PNP discovery are still lacking. We discuss the computational bottlenecks in analyzing PNPs and review recent advances in genome mining, peptidogenomics, and spectral networks that are now enabling the discovery of new PNPs via mass spectrometry. We further describe the connections between these advances and the new generation of software tools for PNP dereplication, de novo sequencing, and identification. From DOI: 10.1039/C5NP00050E
Table of contents
In this Viewpoint article we examine the roles of bacterially produced small molecules in animal defense, development and evolution. From DOI: 10.1039/C4NP00141A
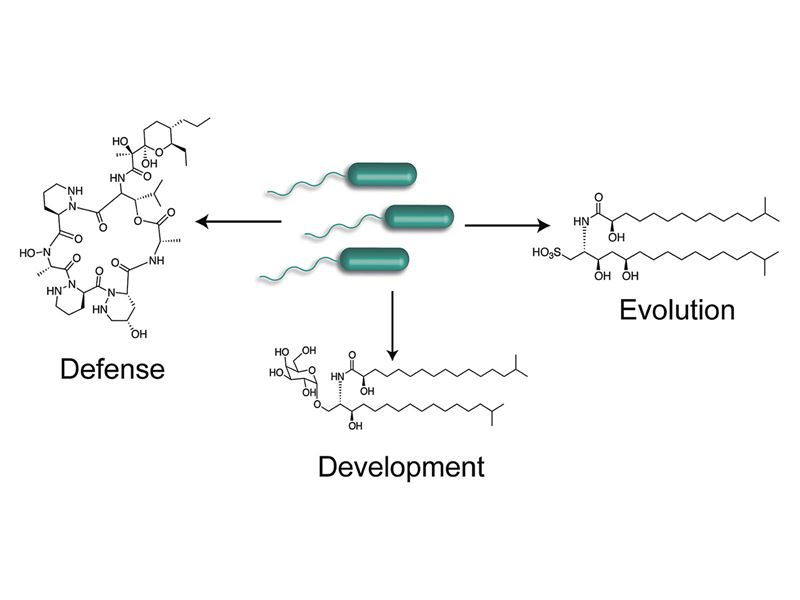
This highlight reviews recent studies of colibactin, a structurally uncharacterized genotoxin synthesised by members of the human gut microbiota. From DOI: 10.1039/C5NP00091B
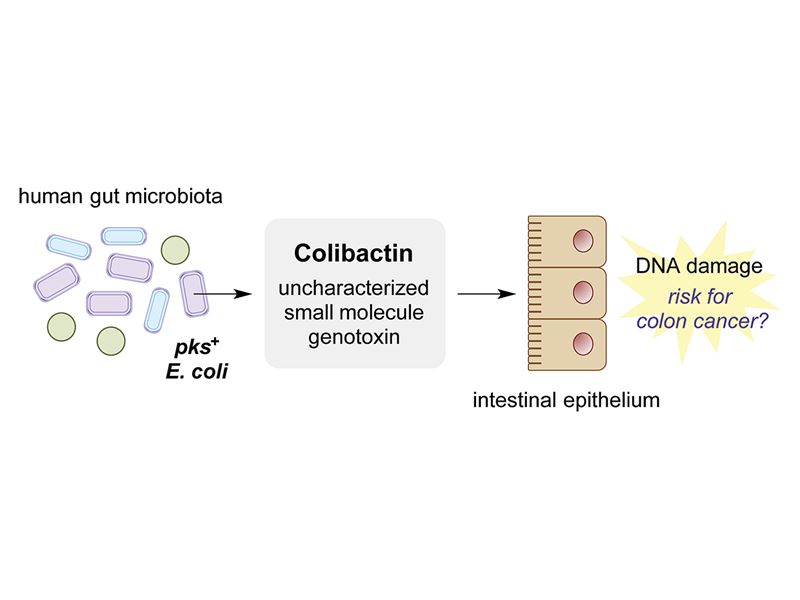
This highlight focuses on one of Nature's key strategies to doubly modify an amino acid during nonribosomal peptide biosynthesis by using a single enzyme, an interrupted adenylation domain. From DOI: 10.1039/C4NP00120F
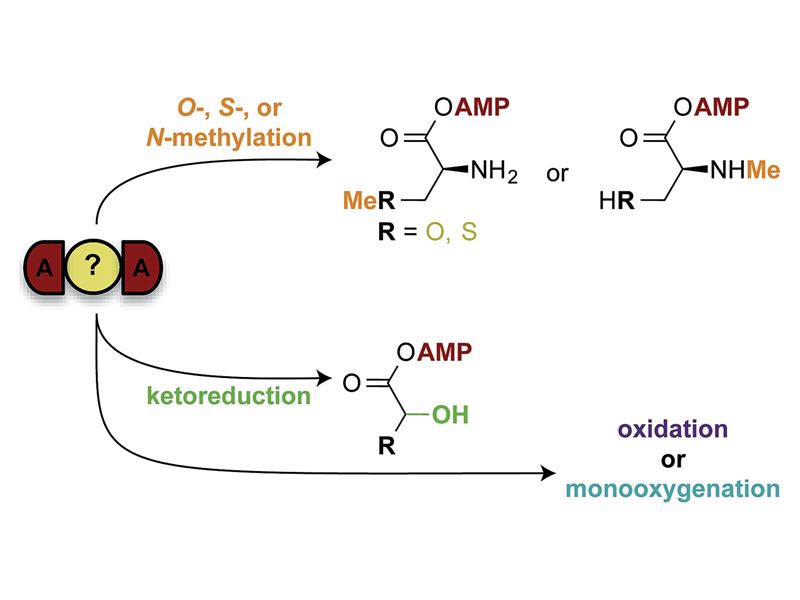
Open access publishing options
Natural Product Reports is a hybrid (transformative) journal and gives authors the choice of publishing their research either via the traditional subscription-based model or instead by choosing our gold open access option. Find out more about our Transformative Journals. which are Plan S compliant .
Gold open access
For authors who want to publish their article gold open access , Natural Product Reports charges an article processing charge (APC) of £2,500 (+ any applicable tax). Our APC is all-inclusive and makes your article freely available online immediately, permanently, and includes your choice of Creative Commons licence (CC BY or CC BY-NC) at no extra cost. It is not a submission charge, so you only pay if your article is accepted for publication.
Learn more about publishing open access .
Read & Publish
If your institution has a Read & Publish agreement in place with the Royal Society of Chemistry, APCs for gold open access publishing in Natural Product Reports may already be covered.
Use our journal finder to check if your institution has an open access agreement with us.
Please use your official institutional email address to submit your manuscript and check you are assigned as the corresponding author; this helps us to identify if you are eligible for Read & Publish or other APC discounts.
Traditional subscription model
Authors can also publish in Natural Product Reports via the traditional subscription model without needing to pay an APC. Articles published via this route are available to institutions and individuals who subscribe to the journal. Our standard licence allows you to make the accepted manuscript of your article freely available after a 12-month embargo period. This is known as the green route to open access.
Learn more about green open access .
Readership information
Academic and industrial scientists working in all areas of natural products research and wider topics including chemical biology, organic synthesis, pharmacology and analytical chemistry.
Subscription information
Natural Product Reports is part of the collection RSC Gold subscription package.
Online only 2024 : ISSN 1460-4752, £1,358 / $2,389
*2022 Journal Citation Reports (Clarivate Analytics, 2023)
**The median time from submission to first decision including manuscripts rejected without peer review from the previous calendar year
***The median time from submission to first decision for peer-reviewed manuscripts from the previous calendar year
****CiteScore™ 2022 available at www.scopus.com/sources
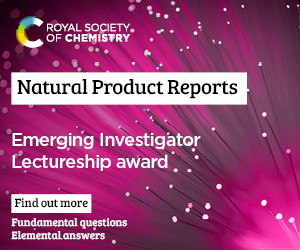
Advertisement
Thank you for visiting nature.com. You are using a browser version with limited support for CSS. To obtain the best experience, we recommend you use a more up to date browser (or turn off compatibility mode in Internet Explorer). In the meantime, to ensure continued support, we are displaying the site without styles and JavaScript.
- View all journals
- Explore content
- About the journal
- Publish with us
- Sign up for alerts
- Review Article
- Published: 14 January 2022
Natural product anticipation through synthesis
- Belinda E. Hetzler ORCID: orcid.org/0000-0002-0372-9126 1 ,
- Dirk Trauner ORCID: orcid.org/0000-0002-6782-6056 1 &
- Andrew L. Lawrence ORCID: orcid.org/0000-0002-9573-5637 2
Nature Reviews Chemistry volume 6 , pages 170–181 ( 2022 ) Cite this article
6472 Accesses
37 Citations
33 Altmetric
Metrics details
- Biosynthesis
- Natural product synthesis
An Author Correction to this article was published on 22 February 2022
This article has been updated
Natural product synthesis remains one of the most vibrant and intellectually rewarding areas of chemistry, although the justifications for pursuing it have evolved over time. In the early years, the emphasis lay on structure elucidation and confirmation through synthesis, as exemplified by celebrated studies on cocaine, morphine, strychnine and chlorophyll. This was followed by a phase where the sheer demonstration that highly complex molecules could be recreated in the laboratory in a rational manner was enough to justify the economic expense and intellectual agonies of a synthesis. Since then, syntheses of natural products have served as platforms for the demonstration of elegant strategies, for inventing new methodology ‘on the fly’ or to demonstrate the usefulness and scope of methods established with simpler molecules. We now add another aspect that we find fascinating, viz. ‘natural product anticipation’. In this Review, we survey cases where the synthesis of a compound in the laboratory has preceded its isolation from nature. The focus of our Review lies on examples where this anticipation of a natural product has triggered a successful search or where synthesis and isolation have occurred independently. Finally, we highlight cases where a potential natural product structure has been suggested as a result of synthetic endeavours but not yet confirmed by isolation, inviting further collaborations between synthetic and natural product chemists.
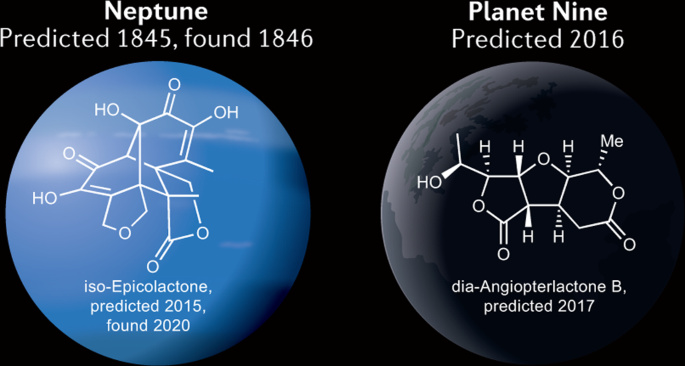
This is a preview of subscription content, access via your institution
Access options
Access Nature and 54 other Nature Portfolio journals
Get Nature+, our best-value online-access subscription
24,99 € / 30 days
cancel any time
Subscribe to this journal
Receive 12 digital issues and online access to articles
111,21 € per year
only 9,27 € per issue
Buy this article
- Purchase on Springer Link
- Instant access to full article PDF
Prices may be subject to local taxes which are calculated during checkout
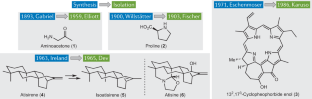
Similar content being viewed by others
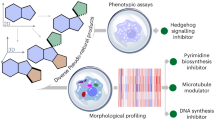
A divergent intermediate strategy yields biologically diverse pseudo-natural products
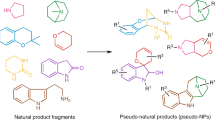
Principle and design of pseudo-natural products
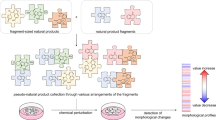
Natural product fragment combination to performance-diverse pseudo-natural products
Change history, 22 february 2022.
A Correction to this paper has been published: https://doi.org/10.1038/s41570-022-00374-w
Nicolaou, K. C. & Snyder, S. A. Chasing molecules that were never there: misassigned natural products and the role of chemical synthesis in modern structure elucidation. Angew. Chem. Int. Ed. 44 , 1012–1044 (2005).
Article CAS Google Scholar
Brown, P. D. & Lawrence, A. L. The importance of asking “how and why?” in natural product structure elucidation. Nat. Prod. Rep. 34 , 1193–1202 (2017).
Article CAS PubMed Google Scholar
Sheehan, J. C. & Henery-Logan, K. R. The total synthesis of penicillin V. J. Am. Chem. Soc. 79 , 1262–1263 (1957).
Kuttruff, C. A., Eastgate, M. D. & Baran, P. S. Natural product synthesis in the age of scalability. Nat. Prod. Rep. 31 , 419–432 (2014).
Baran, P. S. Natural product total synthesis: as exciting as ever and here to stay. J. Am. Chem. Soc. 140 , 4751–4755 (2018).
Trauner, D. Finding function and form. Nat. Prod. Rep. 31 , 411–413 (2014).
Gabriel, S. & Pinkus, G. Zur Kenntniss der Amidoketone. Ber. Dtsch. Chem. Ges. 26 , 2197–2209 (1893).
Gabriel, S. & Colman, J. Zur Kenntniss des Amidoacetons. Ber. Dtsch. Chem. Ges. 35 , 3805–3811 (1902).
Elliott, W. H. Amino-acetone: its isolation and role in metabolism. Nature 183 , 1051–1052 (1959).
Willstätter, R. Synthese der Hygrinsäure. Ber. Dtsch. Chem. Ges. 33 , 1160–1166 (1900).
Article Google Scholar
Fischer, E. & Abderhalden, E. Ober die verdauung einiger Eiweißkörper durch Pankreasfermente. Biol. Chem. 39 , 81–94 (1903).
CAS Google Scholar
Willstätter, R. From My Life: The Memoirs of Richard Willstätter (Plunkett Lake, 2016).
Falk, H., Hoornaert, G., Isenring, H.-P. & Eschenmoser, A. Über Enolderivate der Chlorophyllreihe. Darstellung von 13 2 ,17 3 -Cyclophäophorbid-enolen. Vorläufige Mitteilung. Helv. Chim. Acta 58 , 2347–2357 (1975).
Karuso, P. et al. 13 2 ,17 3 -Cyclopheophorbide enol, the first porphyrin isolated from a sponge. Tetrahedron Lett. 27 , 2177–2178 (1986).
Ocampo, R., Sachs, J. P. & Repeta, D. J. Isolation and structure determination of the unstable 13 2 , 17 3 -Cyclopheophorbide a enol from recent sediments. Geochim. Cosmochim. Acta 63 , 3743–3749 (1999).
Bell, R. A. & Ireland, R. E. The construction of the C/D ring system present in the diterpenoid alkaloids atisine and garryfoline. Tetrahedron Lett. 4 , 269–273 (1963).
Church, R. F., Ireland, R. E. & Marshall, J. A. The stereospecific total synthesis of d1-8β-carbomethoxy-13-oxopodocarpane, a degradation product of phyllocladene. Tetrahedron Lett. 1 , 1–4 (1960).
Zalkow, L. H. & Girotra, N. N. The synthesis of 5a,8,8-trimethyl-3, 10a-ethanoperhydrophenanthrene. Terpenes. VII. J. Org. Chem. 28 , 2037–2039 (1963).
Zalkow, L. H. & Girotra, N. N. Studies in the synthesis of atisine. Terpenes. X. J. Org. Chem. 29 , 1299–1302 (1964).
Kapadi, A. H., Sobti, R. R. & Dev, S. The diterpenoids of Erythroxylon monogynum — V atisirene, isoatisirene and devadarene. Tetrahedron Lett. 6 , 2729–2735 (1965).
Bonneau, N. et al. An unprecedented blue chromophore found in nature using a “chemistry first” and molecular networking approach: discovery of dactylocyanines A–H. Chem. Eur. J. 23 , 14454–14461 (2017).
Fox Ramos, A. E. et al. CANPA: computer-assisted natural products anticipation. Anal. Chem. 91 , 11247–11252 (2019).
Medema, M. H. & Fischbach, M. A. Computational approaches to natural product discovery. Nat. Chem. Biol. 11 , 639–648 (2015).
Article CAS PubMed PubMed Central Google Scholar
Lawrence, A. L. et al. A short biomimetic synthesis of the meroterpenoids guajadial and psidial A. Org. Lett. 12 , 1676–1679 (2010).
Yang, X.-L., Hsieh, K.-L. & Liu, J.-K. Guajadial: an unusual meroterpenoid from guava leaves psidium guajava. Org. Lett. 9 , 5135–5138 (2007).
Collado, I. G., Hanson, J. R. & Macías-Sánchez, A. J. Recent advances in the chemistry of caryophyllene. Nat. Prod. Rep. 15 , 187–204 (1998).
Fu, H.-Z., Luo, Y.-M., Li, C.-J., Yang, J.-Z. & Zhang, D.-M. Psidials A–C, three unusual meroterpenoids from the leaves of Psidium guajava L. Org. Lett. 12 , 656–659 (2010).
Tang, G.-H. et al. Psiguajadials A–K: unusual Psidium meroterpenoids as phosphodiesterase-4 inhibitors from the leaves of Psidium guajava . Sci. Rep. 7 , 1047 (2017).
Article PubMed PubMed Central Google Scholar
Ning, S., Liu, Z., Wang, Z., Liao, M. & Xie, Z. Biomimetic synthesis of psiguajdianone guided discovery of the meroterpenoids from Psidium guajava . Org. Lett. 21 , 8700–8704 (2019).
Chen, Y.-Q., Shen, Y.-H., Su, Y.-Q., Kong, L.-Y. & Zhang, W.-D. Incarviditone: a novel cytotoxic benzofuranone dimer from Incarvillea delavayi Bureau et Franchet. Chem. Biodivers. 6 , 779–783 (2009).
Brown, P. D., Willis, A. C., Sherburn, M. S. & Lawrence, A. L. Total synthesis of incarviditone and incarvilleatone. Org. Lett. 14 , 4537–4539 (2012).
Gao, Y.-P. et al. Incarvilleatone, a new cyclohexylethanoid dimer from Incarvillea younghusbandii and its inhibition against nitric oxide (NO) release. Org. Lett. 14 , 1954–1957 (2012).
Novak, A. J. E. & Trauner, D. Reflections on racemic natural products. Trends Chem. 2 , 1052–1065 (2020).
Kakinuma, K., Hanson, C. A. & Rinehart, K. L. Spectinabilin, a new nitro-containing metabolite isolated from Streptomyces spectabilis . Tetrahedron 32 , 217–222 (1976).
Takahashi, K., Tsuda, E. & Kurosawa, K. SNF4435C and D, novel imimmosuppressants produced by a strain of Streptomyces spectabilis . II. Structure elucidation. J. Antibiot. 54 , 548–553 (2001).
Kurosawa, K., Takahashi, K. & Tsuda, E. SNF4435C and D, novel immunosuppressants produced by a strain of Streptomyces spectabilis . I. Taxonomy, fermentation, isolation and biological activities. J. Antibiot. 54 , 541–547 (2001).
Beaudry, C. M. & Trauner, D. Total synthesis of (−)-SNF4435 C and (+)-SNF4435 D. Org. Lett. 7 , 4475–4477 (2005).
Müller, M. et al. Photochemical origin of the immunosuppressive SNF4435C/D and formation of orinocin through “polyene splicing”. Angew. Chem. Int. Ed. 45 , 7835–7838 (2006).
Lindel, T. Chemistry and biology of the pyrrole–imidazole alkaloids. Alkaloids Chem. Biol. 77 , 117–219 (2017).
Baran, P. S., O’Malley, D. P. & Zografos, A. L. Sceptrin as a potential biosynthetic precursor to complex pyrrole–imidazole alkaloids: the total synthesis of ageliferin. Angew. Chem. Int. Ed. 43 , 2674–2677 (2004).
Northrop, B. H., O’Malley, D. P., Zografos, A. L., Baran, P. S. & Houk, K. N. Mechanism of the vinylcyclobutane rearrangement of sceptrin to ageliferin and nagelamide E. Angew. Chem. Int. Ed. 45 , 4126–4130 (2006).
Endo, T. et al. Nagelamides A–H, new dimeric bromopyrrole alkaloids from marine sponge Agelas species. J. Nat. Prod. 67 , 1262–1267 (2004).
Brastianos, H. C. et al. Exiguamine A, an indoleamine-2,3-dioxygenase (IDO) inhibitor isolated from the marine sponge Neopetrosia exigua . J. Am. Chem. Soc. 128 , 16046–16047 (2006).
Sofiyev, V., Lumb, J., Volgraf, M. & Trauner, D. Total synthesis of exiguamines A and B inspired by catecholamine chemistry. Chem. Eur. J. 18 , 4999–5005 (2012).
Volgraf, M. et al. Biomimetic synthesis of the IDO inhibitors exiguamine A and B. Nat. Chem. Biol. 4 , 535–537 (2008).
Minato, H. & Horibe, I. Structure and stereochemistry of Xanthumin, a stereoisomer of Xanthinin. J. Chem. Soc. Resumed 1965 , 7009–7017 (1965).
Ahmed, A. A., Mahmoud, A. A. & El-Gamal, A. A. A xanthanolide diol and a dimeric xanthanolide from Xanthium species. Planta Med. 65 , 470–472 (1999).
Nour, A. M. M. et al. The antiprotozoal activity of sixteen asteraceae species native to Sudan and bioactivity-guided isolation of xanthanolides from Xanthium brasilicum . Planta Med. 75 , 1363–1368 (2009).
Wang, L. et al. Cytotoxic sesquiterpene lactones from aerial parts of Xanthium sibiricum . Planta Med. 79 , 661–665 (2013).
Feng, J. et al. Enantioselective and collective total syntheses of xanthanolides. Angew. Chem. Int. Ed. 56 , 16323–16327 (2017).
Ren, W. et al. Enantioselective and collective syntheses of xanthanolides involving a controllable dyotropic rearrangement of cis -β-lactones. Angew. Chem. Int. Ed. 51 , 6984–6988 (2012).
Shang, H. et al. Biomimetic synthesis: discovery of xanthanolide dimers. Angew. Chem. Int. Ed. 53 , 14494–14498 (2014).
Brady, S. F., Singh, M. P., Janso, J. E. & Clardy, J. Cytoskyrins A and B, new BIA active bisanthraquinones isolated from an endophytic fungus. Org. Lett. 2 , 4047–4049 (2000).
Jadulco, R. et al. New metabolites from sponge-derived fungi Curvularia lunata and Cladosporium herbarum . J. Nat. Prod. 65 , 730–733 (2002).
Ejiri, H., Sankawa, U. & Shibata, S. Graciliformin and its acetates in Cladonia graciliformis . Phytochemistry 14 , 277–279 (1975).
Yamazaki, H., Koyama, N., Ōmura, S. & Tomoda, H. New rugulosins, anti-MRSA antibiotics, produced by Penicillium radicum FKI-3765-2. Org. Lett. 12 , 1572–1575 (2010).
Ogihara, Y., Kobayashi, N. M. & Shibata, S. Further studies on the bianthraquinones of Penicillium islandicum Sopp. Tetrahedron Lett. 9 , 1881–1886 (1968).
Nicolaou, K. C., Lim, Y. H., Papageorgiou, C. D. & Piper, J. L. Total synthesis of (+)-rugulosin and (+)-2,2′-epi-cytoskyrin A through cascade reactions. Angew. Chem. Int. Ed. 44 , 7917–7921 (2005).
Nicolaou, K. C., Lim, Y. H., Piper, J. L. & Papageorgiou, C. D. Total syntheses of 2,2′-epi-cytoskyrin A, rugulosin, and the alleged structure of rugulin. J. Am. Chem. Soc. 129 , 4001–4013 (2007).
Agusta, A., Ohashi, K. & Shibuya, H. Bisanthraquinone metabolites produced by the endophytic fungus Diaporthe sp. Chem. Pharm. Bull. 54 , 579–582 (2006).
Manzo, E. et al. New γ-pyrone propionates from the Indian Ocean sacoglossan Placobranchus ocellatus . Tetrahedron Lett. 46 , 465–468 (2005).
Cueto, M., D’Croz, L., Maté, J. L., San-Martín, A. & Darias, J. Elysiapyrones from Elysia Diomedea . Do such metabolites evidence an enzymatically assisted electrocyclization cascade for the biosynthesis of their bicyclo[4.2.0]octane core? Org. Lett. 7 , 415–418 (2005).
Miller, A. K. & Trauner, D. Mining the tetraene manifold: total synthesis of complex pyrones from Placobranchus ocellatus . Angew. Chem. Int. Ed. 44 , 4602–4606 (2005).
Wu, Q. et al. Complex polypropionates from a South China Sea photosynthetic mollusk: isolation and biomimetic synthesis highlighting novel rearrangements. Angew. Chem. Int. Ed. 59 , 12105–12112 (2020).
Eccles, R. G. Calycanthine. Drug. Circular Chem. Gaz. 32 , 65 (1888).
Google Scholar
Steven, A. & Overman, L. E. Total synthesis of complex cyclotryptamine alkaloids: stereocontrolled construction of quaternary carbon stereocenters. Angew. Chem. Int. Ed. 46 , 5488–5508 (2007).
Schmidt, M. A. & Movassaghi, M. New strategies for the synthesis of hexahydropyrroloindole alkaloids inspired by biosynthetic hypotheses. Synlett 2008 , 313–324 (2008).
Trost, B. M. & Osipov, M. Recent advances on the total syntheses of the communesin alkaloids and perophoramidine. Chem. Weinh. Bergstr. Ger. 21 , 16318–16343 (2015).
Xu, J.-B. & Cheng, K.-J. Studies on the alkaloids of the calycanthaceae and their syntheses. Molecules 20 , 6715–6738 (2015).
May, J. A. & Stoltz, B. The structural and synthetic implications of the biosynthesis of the calycanthaceous alkaloids, the communesins, and nomofungin. Tetrahedron 62 , 5262–5271 (2006).
Dotson, J. J., Bachman, J. L., Garcia-Garibay, M. A. & Garg, N. K. Discovery and total synthesis of a bis(cyclotryptamine) alkaloid bearing the elusive piperidinoindoline scaffold. J. Am. Chem. Soc. 142 , 11685–11690 (2020).
Hall, E. S., McCapra, F. & Scott, A. I. Biogenetic-type synthesis of the calycanthaceous alkaloids. Tetrahedron 23 , 4131–4141 (1967).
Gavagnin, M., Mollo, E., Cimino, G. & Ortea, J. A new γ-dihydropyrone-propionate from the Caribbean sacoglossan Tridachia crispata . Tetrahedron Lett. 37 , 4259–4262 (1996).
Sharma, P., Lygo, B., Lewis, W. & Moses, J. E. Biomimetic synthesis and structural reassignment of the tridachiahydropyrones. J. Am. Chem. Soc. 131 , 5966–5972 (2009).
Gavagnin, M., Mollo, E. & Cimino, G. Is phototridachiahydropyrone a true natural product? Rev. Bras. Farmacogn. 25 , 588–591 (2015).
Bister, B. et al. Abyssomicin C — A polycyclic antibiotic from a marine Verrucosispora strain as an inhibitor of the p -aminobenzoic acid/tetrahydrofolate biosynthesis pathway. Angew. Chem. Int. Ed. 43 , 2574–2576 (2004).
Nicolaou, K. C. & Harrison, S. T. Total synthesis of abyssomicin C, atrop-abyssomicin C, and abyssomicin D: implications for natural origins of atrop-abyssomicin C. J. Am. Chem. Soc. 129 , 429–440 (2007).
Nicolaou, K. C. & Harrison, S. T. Total synthesis of abyssomicin C and atrop-abyssomicin C. Angew. Chem. Int. Ed. 45 , 3256–3260 (2006).
Keller, S. et al. Abyssomicins G and H and atrop-abyssomicin C from the marine Verrucosispora strain AB-18-032. J. Antibiot. 60 , 391–394 (2007).
Ellerbrock, P., Armanino, N., Ilg, M. K., Webster, R. & Trauner, D. An eight-step synthesis of epicolactone reveals its biosynthetic origin. Nat. Chem. 7 , 879–882 (2015).
Yan, Z. et al. Fused multicyclic polyketides with a two-spiro-carbon skeleton from mangrove-derived endophytic fungus Epicoccum nigrum SCNU-F0002. RSC Adv. 10 , 28560–28566 (2020).
Greshock, T. J. & Williams, R. M. Improved biomimetic total synthesis of d , l -stephacidin A. Org. Lett. 9 , 4255–4258 (2007).
Qin, W.-F. et al. Total synthesis of (−)-depyranoversicolamide B. Chem. Commun. 51 , 16143–16146 (2015).
Xu, X., Zhang, X., Nong, X., Wang, J. & Qi, S. Brevianamides and mycophenolic acid derivatives from the deep-sea-derived fungus Penicillium brevicompactum DFFSCS025. Mar. Drugs 15 , 43 (2017).
Article PubMed Central Google Scholar
Crombie, L. & Ponsford, R. Synthesis of (±)-deoxybruceol. Chem. Commun. Lond. 1968 , 368a (1968).
Crombie, L. & Ponsford, R. Pyridine-catalysed condensation of citral with phloroglucinols, a novel reaction leading to tetracyclic bis-ethers and chromenes. Two-step synthesis of (±)-deoxybruceol. J. Chem. Soc. C Org. 1971 , 788–795 (1971).
Ghisalberti, E. L. et al. Structural studies in the bruceol system. J. Chem. Soc. Perkin Trans. 2 , 583–589 (1981).
Day, A. J., Sumby, C. J. & George, J. H. Biomimetic synthetic studies on the bruceol family of meroterpenoid natural products. J. Org. Chem. 85 , 2103–2117 (2020).
Harada, N. et al. Total synthesis, absolute configuration, and later isolation of (−)-prehalenaquinone, a putative biosynthetic precursor to the marine natural products: halenaquinone and xestoquinone. J. Org. Chem. 59 , 6606–6613 (1994).
Matsuura, B. S., Kölle, P., Trauner, D., de Vivie-Riedle, R. & Meier, R. Unravelling photochemical relationships among natural products from Aplysia dactylomela . ACS Cent. Sci. 3 , 39–46 (2017).
Kotammagari, T. K., Gonnade, R. G. & Bhattacharya, A. K. Biomimetic total synthesis of angiopterlactone B and other potential natural products. Org. Lett. 19 , 3564–3567 (2017).
Nicolaou, K. C., Sanchini, S., Wu, T. R. & Sarlah, D. Total synthesis and structural revision of biyouyanagin B. Chem. Eur. J. 16 , 7678–7682 (2010).
Löbermann, F., Mayer, P. & Trauner, D. Biomimetic synthesis of (−)-pycnanthuquinone C through the Diels–Alder reaction of a vinyl quinone. Angew. Chem. Int. Ed. 49 , 6199–6202 (2010).
Ma, D., Liu, Y. & Wang, Z. Biomimetic total synthesis of (±)-homodimericin A. Angew. Chem. Int. Ed. 56 , 7886–7889 (2017).
Stichnoth, D. et al. Photochemical formation of intricarene. Nat. Commun. 5 , 5597 (2014).
Brown, P. D. & Lawrence, A. L. Total synthesis of millingtonine. Angew. Chem. Int. Ed. 128 , 8421–8425 (2016).
Brown, P. D., Willis, A. C., Sherburn, M. S. & Lawrence, A. L. Total synthesis and structural revision of the alkaloid incargranine B. Angew. Chem. 125 , 13515–13517 (2013).
Purgett, T. J., Dyer, M. W., Bickel, B., McNeely, J. & Porco, J. A. Gold(I)-mediated cycloisomerization/cycloaddition enables bioinspired syntheses of neonectrolides B–E and analogues. J. Am. Chem. Soc. 141 , 15135–15144 (2019).
Novak, A. J. E., Grigglestone, C. E. & Trauner, D. A biomimetic synthesis elucidates the origin of preuisolactone A. J. Am. Chem. Soc. 141 , 15515–15518 (2019).
Li, H., Korotkov, A., Chapman, C. W., Eastman, A. & Wu, J. Enantioselective formal syntheses of 11 nuphar alkaloids and discovery of potent apoptotic monomeric analogues. Angew. Chem. Int. Ed. 55 , 3509–3513 (2016).
Breunig, M., Yuan, P. & Gaich, T. An unexpected transannular [4+2] cycloaddition during the total synthesis of (+)-norcembrene 5. Angew. Chem. Int. Ed. 59 , 5521–5525 (2020).
Nicolaou, K. C., Chen, Q., Li, R., Anami, Y. & Tsuchikama, K. Total synthesis of the monomeric unit of lomaiviticin A. J. Am. Chem. Soc. 142 , 20201–20207 (2020).
Zheng, K., Shen, D., Zhang, B. & Hong, R. Landscape of lankacidin biomimetic synthesis: structural revisions and biogenetic implications. J. Org. Chem. 85 , 13818–13836 (2020).
Su, S., Seiple, I. B., Young, I. S. & Baran, P. S. Total syntheses of (±)-massadine and massadine chloride. J. Am. Chem. Soc. 130 , 16490–16491 (2008).
Strych, S. et al. Biomimetic total synthesis of santalin Y. Angew. Chem. Int. Ed. 54 , 5079–5083 (2015).
Gao, Y. et al. Isolation and biomimetic synthesis of (±)-guajadial B, a novel meroterpenoid from Psidium guajava . Org. Lett. 14 , 5936–5939 (2012).
Yang, B. et al. Asymmetric total synthesis and biosynthetic implications of perovskones, hydrangenone, and hydrangenone B. J. Am. Chem. Soc. 143 , 6370–6375 (2021).
Long, X., Wu, H., Ding, Y., Qu, C. & Deng, J. Biosynthetically inspired divergent syntheses of merocytochalasans. Chem 7 , 212–223 (2021).
Download references
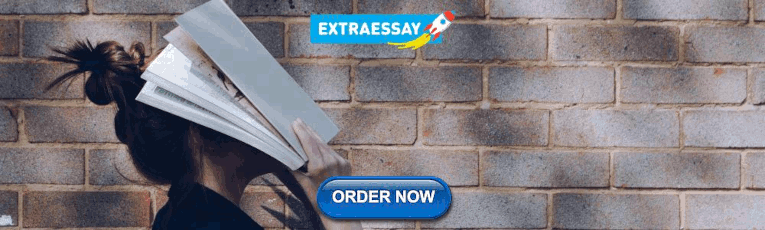
Acknowledgements
B.E.H. thanks New York University for a MacCracken fellowship. The authors thank B. S. Matsuura for helpful discussions. The authors thank B. S. Matsuura, A. J. E. Novak and K.-P. Rühmann for their critical review of the manuscript.
Author information
Authors and affiliations.
Department of Chemistry, New York University, New York, NY, USA
Belinda E. Hetzler & Dirk Trauner
EaStCHEM School of Chemistry, University of Edinburgh, Edinburgh, UK
Andrew L. Lawrence
You can also search for this author in PubMed Google Scholar
Contributions
The authors contributed equally to all aspects of the article.
Corresponding authors
Correspondence to Dirk Trauner or Andrew L. Lawrence .
Ethics declarations
Competing interests.
The authors declare no competing interests.
Additional information
Peer review information.
Nature Reviews Chemistry thanks J. George and the other, anonymous, reviewer(s) for their contribution to the peer review of this work.
Publisher’s note
Springer Nature remains neutral with regard to jurisdictional claims in published maps and institutional affiliations.
Rights and permissions
Reprints and permissions
About this article
Cite this article.
Hetzler, B.E., Trauner, D. & Lawrence, A.L. Natural product anticipation through synthesis. Nat Rev Chem 6 , 170–181 (2022). https://doi.org/10.1038/s41570-021-00345-7
Download citation
Accepted : 13 November 2021
Published : 14 January 2022
Issue Date : March 2022
DOI : https://doi.org/10.1038/s41570-021-00345-7
Share this article
Anyone you share the following link with will be able to read this content:
Sorry, a shareable link is not currently available for this article.
Provided by the Springer Nature SharedIt content-sharing initiative
This article is cited by
Nature-inspired micropatterns.
- Yunhua Wang
- Guoxia Zheng
- Xingcai Zhang
Nature Reviews Methods Primers (2023)
Collective total synthesis of C4-oxygenated securinine-type alkaloids via stereocontrolled diversifications on the piperidine core
- Sangbin Park
- Gyumin Kang
Nature Communications (2022)
Quick links
- Explore articles by subject
- Guide to authors
- Editorial policies
Sign up for the Nature Briefing newsletter — what matters in science, free to your inbox daily.

Advertisement
Recent strategies in nanodelivery systems for natural products: a review
- Open access
- Published: 28 July 2021
- Volume 19 , pages 4311–4326, ( 2021 )
Cite this article
You have full access to this open access article
- Giulia Vanti ORCID: orcid.org/0000-0002-9066-9271 1
4073 Accesses
24 Citations
3 Altmetric
Explore all metrics
Natural products are major molecules for drug discovery due to their structural diversity and their interaction with various biological targets, yet their clinical application is limited by poor water solubility or low lipophilicity, inappropriate molecular size, low dissolution rate and permeation, instability, high metabolic rate and rapid clearance. These issues can be solved by nanomedicine, by improving bioavailability and therapeutic efficacy. Here we review nanocarriers made of polymer or lipid constituents. Specifically, we describe the technological characteristics of each nanosystem, with examples of application to single natural constituents or plant extracts, and possible routes of administration. We report in vitro and in vivo studies and we conclude with the potential advantages of nanodelivery systems in terms of increased stability and solubility, improved biodistribution and efficacy, reduced adverse effects and toxicity.
Similar content being viewed by others
Nanotechnology Applications for Natural Products Delivery
Natural Products and Nanopharmaceuticals
Polymeric Nanocarriers for the Delivery of Phytoconstituents
Avoid common mistakes on your manuscript.
Introduction
Natural products as isolated compounds or extracts of botanical, animal and mineral origin have represented for millennia the only resource to maintain health and to cure or prevent human and animal diseases (Khan et al. 2012 ; Mehta et al. 2015 ). Currently, their role in therapy is still unquestionable and supported by numerous scientific evidences. Natural products also represent the main source of bioactive molecules and play a key role in innovative drug discovery (Newman and Cragg 2020 ) thanks to their enormous structural and chemical variability, which is incomparable to that of any synthetic libraries (Cameron et al. 2011 ; Bilia et al. 2017 ). The extraordinary interest for natural products is mainly due to their capacity to modulate multiple biological targets, activating various signaling or functional pathways and producing a huge therapeutic significance especially for emerging multifactorial and complex diseases, such as cancer, cardiovascular and neurological pathologies (Rodrigues et al. 2016 ). Conversely, many natural products do not possess drug-like characteristics, and their efficacy and clinical use are limited because of physical and/or chemical instability, unsuitable partition coefficient (log P), unfavourable intrinsic dissolution rate or inappropriate molecular size. These unfavourable biopharmaceutical properties often cause low aqueous solubility, poor permeation and absorption through biological membranes and barriers, low biodistribution or rapid metabolism and clearance, resulting in drug plasma levels below the therapeutic concentration and reduced or annulled efficacy. A low bioavailability can be also related to distribution/accumulation of drugs in non-targeted tissues and organs, leading to numerous side effects (Kesarwani et al. 2013 ; Jain & Chella 2020 ) .
Diverse strategies have been used to optimise the bioavailability of natural products (Dragicevic & Maibach 2016 ; Jain & Chella 2020 ; Paroha et al. 2020 ; Saka et al. 2020 ). They are mainly chemical strategies producing semisynthetic compounds or synthetic analogues (Fang & Leu 2006 ; Ita 2016 ), but recently there is an increasing interest in developing appropriate formulations, specifically nanodelivery systems, which present numerous advantages in comparison to conventional drug formulations, such as controlled release kinetics, targeted delivery, enhanced solubility and permeation, increased chemical and physical stability, and extended shelf-life, with a consequent considerable greater clinic effectiveness and less side-effects (Bilia et al. 2017 , 2018 , 2019b ; Chamundeeswari et al. 2019 ).
Drug delivery systems, generally between 50 and 300 nm, up to 1 μm, have nanoscale dimensions ranging, in nature, from the size of a water molecule to the red blood cell diameter, as reported in Fig. 1 . Some nanosized delivery systems have already entered in the clinic because they can offer an advanced approach to optimise the therapeutic efficacy, targeting definite tissues and organs or crossing biological barriers, in addition to improve the safety profile and the compliance of drugs (Bilia et al. 2017 , 2019b ).
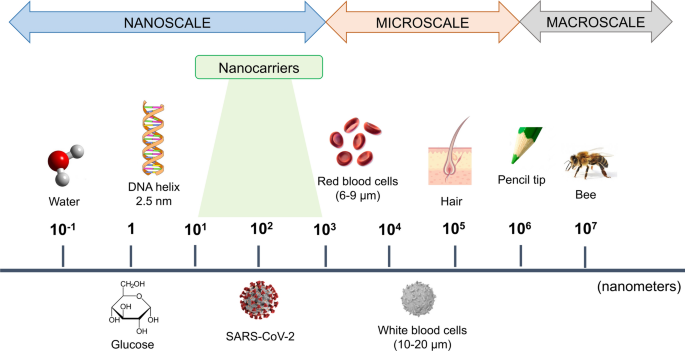
Nanosized drug delivery systems in the scale of nature
This review reports on recent strategies in developing nanodelivery systems for natural products, according to their classification as polymeric-based and lipid-based nanovectors (Fig. 2 ), evidencing their characteristics, advantages and limitations. Specifically, polymeric-based nanocarriers include polymeric nanoparticles, among which nanospheres and nanocapsules, polymeric micelles and dendrimers, whereas lipid nanocarriers include solid lipid nanoparticles, nanostructured lipid carriers, vesicles, nanocochleates, nanoscale emulsions, namely nanoemulsions and microemulsions, and self-microemulsifying drug delivery systems.
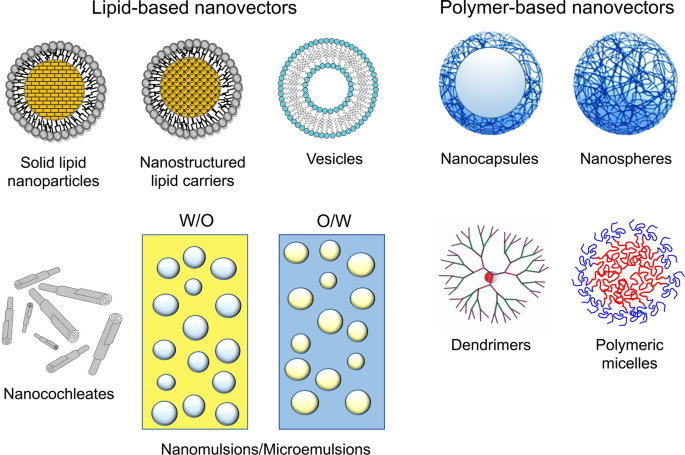
Lipid-based nanovectors, including solid lipid nanoparticles, nanostructured lipid carriers, vesicles, nanocochleates and micro/nanoemulsions. Polymeric-based nanovectors, including nanospheres, nanocapsules, dendrimers and polymeric micelles. W: water, O: oil
This article is an abridged version of the chapter by Anna Rita Bilia, Vieri Piazzini, Maria Camilla Bergonzi ( 2020 ) Nanotechnology Applications for Natural Products Delivery. Sustainable Agriculture Reviews 44 (pp. 1-46). Springer, Cham.
Polymeric nanocarriers
Polymeric nanocarriers include nanoparticles (nanocapsules and nanospheres), dendrimers and polymeric micelles (Fig. 2 ), made of natural, semi-synthetic or synthetic polymers, selected on the basis of their biodegradability, biocompatibility and surface characteristics, with the aim to produce novel and safe carriers having a controlled and targeted drug delivery. Natural polymers originate from bacteria, fungi, animals, plants, and are mainly represented by polysaccharides (principally chitosan, cellulose, alginic acid, carrageenan, arabic gum, pectin, starch, xanthan, gellan) (Divya et al. 2018 ; Parhi 2020 ) and proteins (albumin, casein, gelatin, soy protein hydrolysate) (Elzoghby et al. 2012 ). Synthetic polymers include poly-cyanoacrylate alkyl esters, polylactic acid, poly (N-vinyl pyrrolidone), polyvinyl alcohol, polyglycolic acid, polylactic glycolic acid (Bilia et al. 2017 , 2018 , 2019b ; Kumar et al. 2019 ), whereas semi-synthetic polymers are obtained by modification of natural polymers with synthetic polymers or synthetic chemicals by different methods, such as blending, crosslinking and grafting (Sithole et al. 2017 ).
Polymeric nanoparticles
Generally, polymeric nanoparticles consist of nanocapsules and nanospheres with dimensions ranging from 100 to 500 nm. In nanocapsules the drug is loaded in the central cavity delimited by the polymeric membrane, whereas in nanospheres the drug is distributed inside the polymeric matrix. Nanoparticles can be used for different administration routes, including the oral and parenteral ones, and they can strongly increase drug bioavailability, as well as allow sustained release of the loaded drug or selectively cross barriers, such as the blood-brain barrier (Guccione et al. 2017 ; Ahuja et al. 2020 ).
Specifically, nanoparticles for parenteral administration, formulated using a mixture of polymers, namely poly lactide-co-glycolide and monomethoxy polyethylene glycol, were loaded with ginkgolides and bilobalide, the active constituents of Ginkgo biloba L., with an encapsulation efficiency of about 79% and a drug loading of ca. 12 mg per 150 mg of polymer. The mean diameter of developed nanoparticles was ca. 123 nm, while the zeta potential was ca. -30 mV. The half-life time of the gingko compounds was moreover considerably increased by loading into the polymeric nanoparticles (Han et al. 2012 ).
Polymeric nanoparticles are also able to modify the pharmacokinetic profile of curcumin after oral administration. Nanoparticles were prepared using polylactic polyglycolic acid, enhancing the relative oral bioavailability of curcumin by 563% compared to the unformulated curcumin. The increased bioavailability was due to the inhibition of P-gp-mediated efflux, allowing to rise permeability and absorption of drug in the blood stream (Xie et al. 2011 ).
Polymeric nanoparticles have been also evaluated for their capability to cross blood-brain barrier after systemic administration in rats. Specifically, nanoparticles based on poly ethylcyanoacrylate, and coated with polysorbate 80, were loaded with salvianolic acid B and evaluated for their biodistribution (Grossi et al. 2017 ). Salvianolic acid B represents the main constituent of Salvia miltiorrhiza L. and it is able to prevent nervous degeneration in numerous animal models with different biological mechanisms (Bonaccini et al. 2015 ), however its poor chemical stability and low bioavailability severely limit its therapeutic use. The study included intracerebral injection of nanoparticles in healthy rats, in order to observe the distribution of nanoparticles within the injected hemisphere, where they mainly interacted with microglial cells, probably involved in their clearance by phagocytosis. Nanoparticles were furthermore found to be no toxic by chronical administration in C57/B6 mice. The encapsulation efficacy of salvianolic acid B was about 99%, whereas the loading capacity was about 53%. The mean diameter of nanoparticles resulted less than 300 nm and zeta potential was about -8.4 mV. The nanoparticles moreover showed a modified release of salvianolic acid B, during 8 h (Grossi et al. 2017 ).
Recently, albumin nanoparticles, prepared using two different cross-linking methods, the chemical and the thermal one, were investigated as alternative approach to cross the blood-brain barrier after intravenous and intraperitoneal administration in healthy rats. Nanoparticles were found to be safe by behavioural tests on rats, including tests on locomotor and explorative activities, as well as on the cognitive function (Bergonzi et al. 2016 ). Also, nanoparticles did not induce any inflammatory reaction. In a further investigation, developed albumin nanoparticles were thereby loaded with the characteristic terpenoid andrographolide, the main active constituent of Andrographis paniculate L., whose properties in the neurodegenerative pathologies are well known (Casamonti et al. 2019a ). The ability of albumin nanoparticles in crossing the blood-brain barrier was compared with that of poly ethylcyanoacrylate nanoparticles (Guccione et al. 2017 ). The studies were carried out using an in vitro model of blood–brain barrier based on human cerebral microvascular endothelial cell line (hCMEC/D3). The authors found that free andrographolide did not cross the endothelial cell line, giving consistent results with the in silico studies; poly ethylcyanoacrylate nanoparticles were able to cross the barrier, but they provisionally disordered the integrity of the barrier model, whereas albumin nanoparticles permeated the barrier model, preserving the integrity of the barrier (Guccione et al. 2017 ).
In a very recent study, andrographolide-loaded albumin nanoparticles were evaluated after parental administration for their distribution and pharmacological effects in brain using TgCRND8 mice, a mouse model of Alzheimer's disease. The nanoparticles had mean size of about 160 nm and a zeta potential of about -25 mV. Encapsulation efficiency was greater than 99%. Nanoparticles administered to TgCRND8 mice were evaluated using the step down inhibitory avoidance test and it was observed that nanoparticles significantly enhanced ( p <0.0001) the efficacy of loaded drug in TgCRND8 mice, which reached comparable performances to those obtained in wild type mice. In the object recognition test, both animals treated and untreated with andrographolide-loaded albumin nanoparticles showed no deficiencies in locomotor activity, exploratory activity and directional movement towards objects, in addition to no cognitive impairments ( p <0.0001). Albumin nanoparticles loaded with the fluorescent probe, fluorescein sodium salt, were traced in the brain parenchyma of TgCRND8 mice, after intravenous and intraperitoneal administration. In addition, the immunofluorescent analyses revealed the distribution of NAF-loaded nanoparticles both in the pE3-Aβ plaque surroundings and inside the pE3-Aβ plaque, evidencing their ability to cross the blood-brain barrier and penetrate both undamaged and damaged brain tissues (Bilia et al. 2019a ).
Reviewed studies are some examples of the wide research on polymeric nanoparticles as future nanomedicines for the treatment of various diseases. Polymeric nanoparticles were found to be viable nano-sized delivery system in loading diverse type of natural products, such as ginkgolides, bilobalide, curcumin, salvianolic acid B and andrographolide, for oral and parenteral administration, thanks to the high biocompatibility of constituents, resulting a promising strategy for the translation in clinical therapy.
Polymeric micelles
Polymeric micelles are nanovectors, ranging from 20 to 200 nm, formed by the aggregation of amphiphilic block copolymers made of hydrophobic and hydrophilic monomer units. Micelles spontaneously form by self-association, when the concentration of the monomer unit reaches the critical micellar concentration, similarly to the formation of surfactant-based micelles (Fig. 3 ). Polymeric micelles are very versatile, stable, safe and low cost nanovectors, and they can be used for almost all the administration routes (Bilia et al. 2017 , 2019b ).
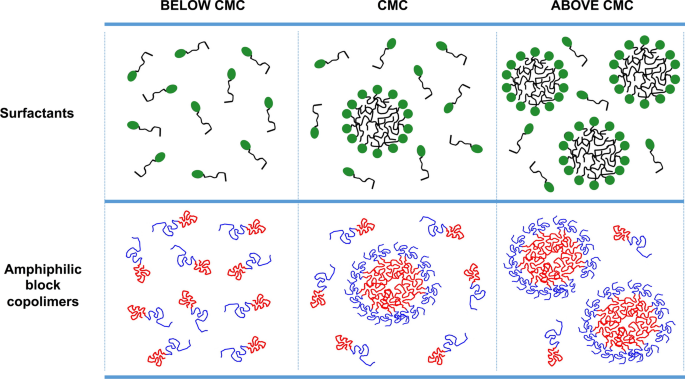
Polymeric micelles are formed by the aggregation of amphiphilic block copolymers, made of hydrophobic and hydrophilic monomer units. Micelles spontaneously form by self-association, when the concentration of the monomer unit reaches the critical micellar concentration (CMC), similarly to the formation of surfactant-based micelles
A study on polymeric micelles loaded with curcumin was carried out to evaluate the inhibition of human brain glioblastoma proliferation. These micelles (about 142 nm) were prepared with an amphiphilic block copolymer synthetized by esterification of polyethylene glycol 400 (hydrophilic portion) and oleoyl chloride (lipophilic portion). In addition, their effects on regular human fibroblastic cells (HFSF‑PI3) and adult human bone marrow stromal cells were investigated. Glioblastoma cell viability was not affected by unformulated curcumin. By contrast curcumin-loaded micelles significantly ( p < 0.001) inhibited the proliferation of glioblastoma, meanwhile the same dosages (20, 25, 30 and 35 μM) of micelles did not affect regular fibroblastic cells and stromal cells, demonstrating a very high selectivity (Tahmasebi Mirgani et al. 2014 ).
Another study focused on polymeric micelles made of soluplus (polymer of polyvinyl caprolactam, polyethylene glycol and polyvinyl acetate) and polymeric mixed micelles made of soluplus plus vitamin E polyethylene glycol 1000 succinate (TPGS) in 20:1 gravimetric ratio, for the oral administration of silymarin. Increasing amounts of the active constituent (from 0.5 to 4 mg/mL) were loaded inside micelles. Up to 3 mg/mL, silymarin-loaded micelles maintained similar sizes (about 60 nm), low polydispersity index (≤ 0.1) and high encapsulation efficiency (> 92%). Apparent solubility of silymarin in the micelles was considerably increased, up 6-fold, and the nanovectors eluded silymarin degradation in the gastrointestinal tract. The in vitro permeation assay, by parallel artificial membranes mimicking the intestinal membrane, confirmed the enhancement of passive diffusion of silymarin when loaded in micelles, whereas the transport studies with Caco 2 cell lines, besides demonstrating that both types of micelles entered into Caco-2 cells via energy-dependent mechanisms, also indicated that polymeric mixed micelles enhanced the permeability of silymarin compared to polymeric micelles and unformulated extract (Piazzini et al. 2019 ). In the last years, nano-sized micelles have obtained increasing interest for diagnosis and treatments of many diseases, culminated with the approval by the Food and Drug Administration of the first micelle formulation of paclitaxel for the treatment of breast, ovarian and lung cancer. The lipophilic core increases the solubility of poorly water-soluble molecules, such as curcumin and silymarin, as described in reported studies, and provides a controlled drug release, while the hydrophilic shell protects the encapsulated drug from the external medium, resulting in long circulation properties and increased drug bioavailability.
The name “dendrimer” comes from the Greek word “ δένδρον” (dendron), meaning “tree”. In fact, dendrimers are self-assembling globular structures with a central core surrounded by branched polymers. Their size range (from some nanometers up to 10-20 nm) is modulated by varying dendrimer generation numbers (for instance G0, G1, G2, Fig. 4 ). Different surface properties can be obtained according to the linked functional groups. Drugs can be chemically linked to the superficial moieties or encapsulated into the internal cavities. Amine derivatives (poly(amidoamine) and poly(propylene imine)) are used for the preparation of dendrimers, whereas other polymers are used to functionalise their surface, such as the poly-L-glutamic acid and the polyethylenglycol (Bilia et al. 2017 , 2019b ).
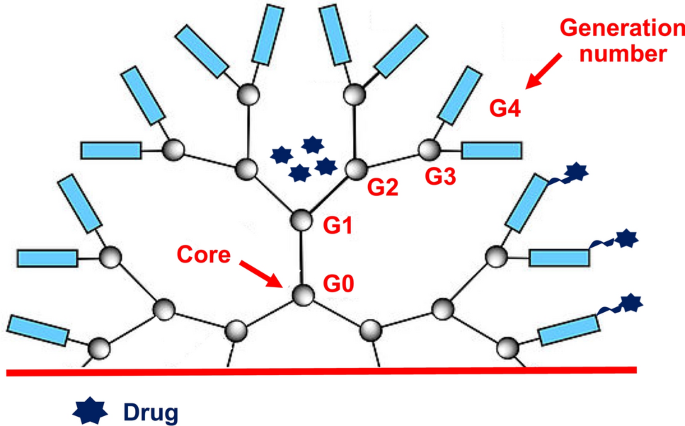
Dendrimers are self-assembling nanoparticles with a central core surrounded by branched polymers. Their size range is modulated by varying dendrimer generation numbers, for instance G0, G1, G2. Different surface properties can be obtained according to the different linked functional groups. Drugs can be chemically linked to the superficial moieties or encapsulated into the internal cavities
Recently, dendrimers with spherical shape and about 150 nm diameter were prepared using polyamidoamine and loaded with curcumin, in order to increase its solubility and bioavailability. The developed dendrimers had no cytotoxic effects in breast cancer MCF-7 cells and curcumin solubility was enormously increased; it was found to be up to ca. 415 times more soluble than unformulated curcumin (Falconieri et al. 2017 ).
Dendrimers are increasingly fascinating researchers and they hold a promising future not only in delivery of drugs and phytochemical bioactive compounds, but also in diagnosis and management of diseases. Multistep synthesis sometimes gives low yield which can be overcome by variation of the chemical method of preparation. A certain cytotoxicity, which depends on generation to which dendrimers belong and on nature of functional groups on their surface, was also observed, but can be managed by modifications of structure and constituents of dendrimers (Chis et al. 2020 ). Overall, their unique properties such as nanoscale uniform size, high degree of branching, polyvalency, water solubility, available internal cavities and convenient synthesis approaches make them promising agents for biological and drug delivery applications (Sherje et al. 2018 ).
Lipid nanocarriers
Lipid-based nanovectors are prepared using natural and synthetic fatty acids, fatty alcohols, waxes, oils and fats, steroid, amphipathic steroids (Bilia et al. 2019b ). These nanovectors include nano-scale emulsions (microemulsions and nanoemulsions), self-microemulsifying drug delivery systems, solid lipid nanoparticles, nanostructured lipid carrires and vesicles, which can be converted in nanocochleates (Fig. 2 ) (Bilia et al. 2019b ).
Microemulsions and nanoemulsions
Microemulsions and nanoemulsions are prepared using an oily phase, an aqueous phase and surfactants agents (McClements et al. 2012). The resulting system is optically transparent with droplets (oil in water or water in oil) of nano-sized dimensions (10-100 nm). However, the two systems are significantly different (Fig. 2 ). In fact, nanoemulsions have a spontaneous propensity to separate in the two initial immiscible phases and need high energy, generally by a microfluidic or ultrasonic approach, to be formed (Tayeb at al. 2018). By contrast, microemulsions can generate spontaneously when all the components are mixed together and they are optical isotropic, homogeneous and thermodynamically stable transparent systems (Tartaro et al. 2020 ). They can be described as oil in water emulsions, water in oil emulsion or biphasic bicontinuos systems (Fig. 5 ), (Bilia et al. 2017 , 2019b ). In many cases, self-microemulsifying drug delivery systems can be formulated as microemulsion precursors, since the latter can form as soon as they come in contact with the external phase (Kang et al. 2004 ). Very interesting, microemulsions and nanoemulsion can be used for loading isolated natural compounds or complex mixtures of natural products (Kumar et al. 2018 ), such as extracts and essential oils (Barradas et al. 2020 ). In addition, all the administration routes are suitable for these versatile systems (Bilia et al. 2017 , 2019b ).
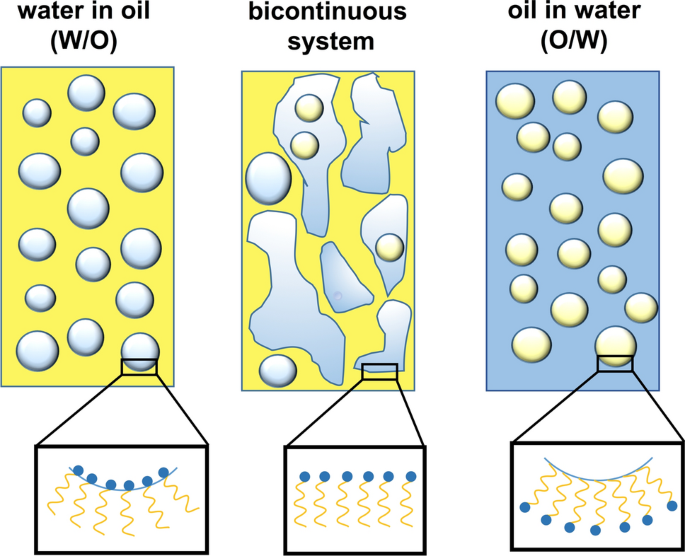
Microemulsions can be water (W) in oil (O), bicontinuos systems or oil in water
An oil-in-water nanoemulsion containing capsaicin was formulated with olive oil (oily phase), Tween 80, Span 80 (surfactants), ethanol (co-surfactant) and water, in order to treat pain and inflammatory disorders. The internal phase droplets had very small sizes ( ca. 14 nm) and did not show coalescence phenomena for periods longer than 8 months, at 4°C and 45°C. The obtained nanoemulsion was formulated as gel (Carbopol®) and cream (cholesterol, liquid paraffin, soft paraffin, cetyl alcohol and beeswax) containing 0.075% capsaicin. After topical application, the capsaicin-formulation showed significant anti-inflammatory activity in rat paw oedema and high resistance to pain signals (Ghiasia et al. 2019 ).
Three microemulsions, made of Cremophor EL, lecithin, Tween 20 and Tween 80 as surfactants, and containing wheat germ oil, olive oil and vitamin E as oily phase, were tested in vitro for permeation evaluation of curcumin after oral administration, using the parallel artificial membrane permeability assay. The best performance, with a curcumin permeation of about 70%, was obtained by the formulation prepared with vitamin E (3.3 g/100 g), Tween 20 (53.8 g/100 g), ethanol (6.6 g/100 g) and water (36.3 g/100 g), (Bergonzi et al. 2014 ).
Recently, Piazzini and coworkers (Piazzini et al. 2017a , 2017b ) successfully tested innovative oil-in-water nanoemulsions, with droplet sizes ranging from 10 to 20 nm, in order to improve the oral absorption of extracts of Vitex agnus-castus L. and Silybum marianum L. Silybum marianum L. extract (4% w/w ) was formulated using Labrasol, Cremophor EL, Labrafil and water. Vitex agnus-castus L. extract (6% w/w ) was instead formulated using triacetin, Labrasol, Cremophor EL and water. The studies extensively proved that nanoemulsions improved the permeation of the characteristic constituents of the extracts, when compared with the unformulated extracts.
In a further study, a microemulsion based on Labrasol, Cremophor EL, glycerol and water was formulated and loaded with green tea catechins and caffeine. The microemulsions well-preserved the antioxidant properties of the loaded constituents and the formulation was safe for mammalian cells (Gupta et al. 2019).
Another microemulsion, composed of triacetin, Tween 20, Labrasol and water, was loaded with Salicis cortex L. extract (40 mg/mL). Droplet sizes were about 40 nm and the solubility of the characteristic constituents of Salicis cortex L. was improved with respect to the unformulated extract (between 2 and 3.6 times more). In vitro tests, by parallel artificial membranes and Caco-2 cells, evidenced an enhanced permeation of Salicis cortex L. constituents when the extract was loaded in microemulsion (Piazzini et al. 2018a ).
A self-microemulsifying drug delivery system, formulated with castor oil, Cremophor EL and 1,2-propanediol, was loaded in sustained-release pellets, for the oral administration of puerarin. The pharmacokinetic profile and the bioavailability of loaded drug was assessed in beagle dogs. The absolute bioavailability of puerarin loaded in the innovative formulation increased 2.6-fold, when compared with that obtained using a conventional formulation, while the relative bioavailability was found to be 259.7% (Zhang et al. 2012a).
Another study was focused on two microemulsions and two self-microemulsifying drug delivery systems for the oral administration of a complex commercial blend based on carbon dioxide extract of Serenoa repens L. (saw palmetto). Stability of the formulations was assessed in simulated gastric and intestinal fluids. The in vitro parallel artificial membrane permeation assay furthermore indicated a greater mucosal permeation for blend based on saw palmetto extract formulated in the nanosystems compared to the raw commercial blend or the single saw palmetto extract. Permeation values of developed nanoemulsions and self-microemulsifying drug delivery systems were between 30% and 70% (Guccione et al. 2018 ).
Recently, an olive extract from Tuscan unripe olives ( Olea europaea L.), characterized by oleuropein ( ca. 31%), ligstroside ( ca. 3%) and verbascoside ( ca. 2.5%) was loaded at the concentration of 35 mg/mL in a microemulsion, for oral administration. The developed formulation, composed of Capryol 90, Cremophor EL, Transcutol and water, was stable at +4°C for three months. The permeability of phenol constituents, evaluated using the parallel artificial membrane permeation assay, was more than 2 times higher compared to that of unformulated olive extract. The transport studies carried out with Caco-2 cells moreover confirmed the increased permeation of the formulated extract (P app of 26.99 ± 0.45 × 10 -6 cm/s versus 16.14 ± 0.05 × 10 -6 cm/s for the unformulated extract), (Cecchi et al. 2020 ).
Isolated natural products (capsaicin, curcumin, puerarin), hydroalcoholic extracts ( Vitex agnus-castus L., Silybym marianum L., Salicis cortex L., Olea europaea L.) and carbon dioxide extracts ( Serenoa repens L.) were successfully formulated in microemulsions or nanoemulsions or self-microemulsifying drug delivery systems, obtaining increased solubility or increased permeability through artificial membranes mimicking intestinal mucosa or improved bioavailability. Over the last decades, nano-sized emulsions have been areas of study for a wide range of applications, from food science to cosmetics and drug delivery. In order to facilitate the translation of nano-sized emulsion from research laboratories to the pharmaceutical market, a careful selection of constituents must be done, with regard to safety concerns including hypersensitivity. The selection of biocompatible and approved excipients is a prerequisite for any future pharmaceutical application. In addition, formulation challenges such as ease of synthesis at large scale, storage and stability must be addressed in view of the therapeutic application (Tayeb at al. 2018; Tartaro et al. 2020 ).
Solid lipid nanoparticles and nanostructured lipid carriers
Solid lipid nanoparticles and nanostructured lipid carriers (Fig. 2 ) are colloidal systems with a lipid matrix stabilized by surfactants. They have dimensions ranging from 50 to 1000 nm, and they are suitable for all the administration routes (Mehnert and Mäder 2012 ). These nanovectors are highly biocompatible, biodegradable, safe and very stable. Typically, the lipid core of solid lipid nanoparticles consists of glycerides, fatty acids, fatty alcohols and waxes, which are solid at room temperature and generally recognized as safe ingredients. Nanostructured lipid carriers represent an evolution of solid lipid nanoparticles, obtained by modification of the lipid core structure, adding a liquid lipid at room temperature (Das and Chaudhury 2010 ). Due to the generation of an imperfect matrix, nanostructured lipid carriers are characterized by higher entrapment efficiency and more stable drug loading (Poonia et al. 2016 ; Bilia et al. 2019b ). The liquid lipids are generally represented by medium chain triglycerides and edible oils, including corn oil, soybean oil, sunflower oil, vitamin E (Tamjidi et al. 2013 ).
Solid lipid nanoparticles based on stearic acid, lecithin and Myrj 52 were loaded with curcumin and investigated for their efficacy in an allergic rat model of asthma by intraperitoneal injection. The nanovectors had dimensions of ca. 190 nm, with 75% drug entrapment efficiency. Enhanced plasmatic and tissue curcumin concentrations, especially in lung and liver, were obtained with the solid lipid nanoparticles when compared with unformulated curcumin. The nanovectors inhibited airway hyper-responsiveness and cell infiltration, in addition to suppress the expression of interleukin-4 and interleukin-13 (Wang et al. 2012 ). Since curcumin has a wide spectrum of biological properties, but it is poorly soluble in water with consequent low oral bioavailability, it has been also formulated in solid lipid nanoparticles for oral administration by Righeschi and coworkers (Righeschi et al. 2016 ), with the aim to enhance its permeation through gastrointestinal mucous membranes. These solid lipid nanoparticles, prepared with Compritol and GRAS ingredients, and loaded with curcumin, had average diameter of about 300 nm, drug loading capacity of ca. 1.60% and drug entrapment efficiency of 80%. They showed high stability over one month of storage at +4°C and significantly improved the permeation of curcumin by parallel artificial membranes.
Other interesting active molecules has been delivered by solid lipid nanoparticles, in order to improve their oral bioavailability. An example is andrographolide, formulated in nanoparticles based on compritol 888 ATO, glyceryl monostearate, lecithin and Tween-80, with entrapment efficiency of about 91% and drug loading of about 3.5%. Its bioavailability and antihyperlipidemic activity were improved in comparison to the unformulated andrographolide, by increasing its solubility and stability in the intestine and by changing its transport mechanism (evaluated in Caco-2 cell line), (Yang et al. 2013 ). Andrographolide was also formulated in stealth solid lipid nanoparticles based on compritol 888 ATO and Brij 78, for parenteral administration and brain delivery. Developed nanoparticles remained unchanged in presence of human serum albumin and plasma, in addition to be chemically and physically stable over 1 month of storage. The ability of these nanovectors to cross the blood-brain barrier was assessed using parallel artificial membranes and hCMEC/D3 cells, a well-establish in vitro model of human blood-brain barrier, in order to predict andrographolide absorption by transcellular passive diffusion. The permeation of andrographolide loaded in nanoparticles was increased with respect to the unformulated andrographolide. Furthermore, after intravenous administration in healthy rats, fluorescent solid lipid nanoparticles were detected in brain parenchyma outside the vascular bed, confirming their ability in vivo to cross the blood-brain barrier (Graverini et al. 2018 ).
Silymarin, extracted from seeds of Silybum marianum L., has been used for decades as hepatoprotectant and, recently, it has been proposed for beneficial effects in type 2 diabetes patients. However, silymarin is poorly soluble in water, with limited oral bioavailability. Thus, in order to enhance its solubility and intestinal absorption, Piazzini and coworkers (Piazzini et al. 2018b ) developed nanostructured lipid carriers using stearic acid, capryol 90 and Brij S20. Obtained nanovectors were stable after incubation in simulated gastric and intestinal fluids. Evaluation of permeability of formulated silymarin, by parallel artificial membrane permeability assay and Caco-2 cells, evidenced an increased uptake via active transport mechanisms. Other authors also investigated nanostructured lipid carriers made of glyceryl monostearate and oleic acid, for the hepatic delivery of silymarin by oral route. In vivo studies in rats highlighted that silymarin loaded in the nanovectors was absorbed through the lymphatic system after oral gavage and its relative bioavailability was 2-fold higher compared to the suspension of silymarin. In addition, a significant amount of silymarin reached the liver 2 h after the administration (Chaudhary et al. 2015 ).
However, the nanostructured lipid carriers can be formulated also for dermal application. Nanoparticles made of soy lecithin, glyceryl monostearate, stearic acid, and medium chain triglycerides, for instance, were loaded with quercetin and evaluated as topical delivery system. The average particle sizes were ca. 215 nm, the drug loading was about 3% and the encapsulation efficiency was about 90%. In vitro permeation studies, using mouse skin, evidenced the enhanced permeation of quercetin with respect to the unformulated quercetin, whereas in vivo drug distribution experiments evidenced quercetin retention in epidermis and dermis (Chen-Yu et al. 2012 ).
Solid lipid nanocarriers were developed in the early ‘90s to overcome the weaknesses of traditional colloidal carriers, like polymeric nanoparticles and liposomes. Reviewed researches deal with the formulation of natural products, such as curcumin, andrographolide, silymarin and quercetin, recognized as pharmacological active substances, but poorly soluble in water and with low bioavailability. The good biocompatibility of lipid excipients makes it possible to use solid lipid nanocarriers in several delivery routes, including parenteral, oral, and topical, as described. However, despite the increased research interests on these drug carriers, limited pharmaceutical products containing solid lipid nanoparticles are on the market. More knowledge are required concerning the interactions between excipients and biological tissues of the target sites, as well as the fate of solid lipid nanocarriers in the systemic distribution. Additionally, an easy production on large-scale may also advance the commercializing process (Mishra et al. 2018 ; Mu et al. 2018 ; Scioli Montoto et al. 2020 ).
Lipid vesicles
Vesicle preparation was firstly reported in 1965 using natural phospholipids. Vesicles can be obtained using amphiphilic molecules, represented by natural or synthetic phospholipids (liposomes) or non-ionic surfactants (niosomes), (Fig. 2 ). Vesicles are generally spherical with sizes ranging from 50 nm to several microns and having a primary role in affecting their biodistribution and pharmacokinetics. Cholesterol is commonly added as fluidizing of the bilayer, although it does not participate in the bilayer formation (Bilia et. al 2019b ; Bozzuto et al. 2015 ). They are characterized by high biocompatibility and biodegradability, high versatility because they are suitable for all the administration routes and can be loaded with both hydrophilic and hydrophobic drugs with resulting enhanced solubility, permeability, stability, improved bioavailability, controlled release and decreased toxicity of drugs. Vesicles also present ease surface modification to obtain passive or active targeting delivery or prolonged circulation and life time. Major limit is the possible drug leakage, due to the release from the vesicles, together with the low stability of the colloid (Bilia et al. 2014a , 2014b , 2017 , 2019b ).
Conventional liposomes
Conventional liposomes are first-generation drug delivery systems but still represent extremely relevant carriers. Their usefulness is also related to their versatility and wide-ranging use because of their flexibility in being formulated in a broad range of pharmaceutical dosage forms, including (colloidal) solutions, aerosol, semi-solid or solid forms. Accordingly, many approaches of formulations have been applied both to isolated natural products, extracts and essential oils (Bilia et al. 2017 , 2019b ).
An in vivo study on verbascoside-loaded liposomes demonstrated that the liposomal formulation improved the chemical stability and the therapeutic performance of the active molecule. Liposomes, with an average diameter around 120 nm and 83% of encapsulation efficiency, were tested in two animal models of neuropathic pain and the performance of the liposomal verbascoside was compared with that of unformulated drug. Verbascoside possesses, in fact, various pharmacological activities for human health, including antioxidant, anti-inflammatory and antineoplastic properties in addition to numerous wound healing and neuroprotective properties. The paw pressure test was carried out administering verbascoside-loaded liposomes in chronic constriction injury rats by intraperitoneal injection at the dosage of 100 mg/kg, and presented a prolonged antihyperalgesic effect for loaded-verbascoside in comparison to the free drug. The effect has onset 15 min after the injection and persisted for 60 min (Isacchi et al. 2016 ).
Naringenin is a flavonoid with diverse pharmacological activities. However, upon ingestion it could be degraded into an active aglycone by the action of certain intestinal bacterial enzymes and the formulation in liposomes was proposed to stabilize the molecule and enhance its bioavailability, in addition to increase its water solubility. Developed liposomes had dimensions suitable for the oral route ( ca. 70 nm), whereas in vivo studies in mice showed an increased area under the curve, indicating a correspondent enhanced bioavailability (about 14-fold) of naringenin after oral administration. Also, studies of tissue distribution evidenced a predominant accumulation of liposomal naringenin in the liver, with respect to the free drug (Wan et al. 2017 ).
As conventional liposomes are at times unstable when exposed to endogenous chemicals and enzymes in the gastrointestinal environment, an interest study reports the effect of different pluronics (F127, F87 and P85), triblock copolymers many of which approved for use as food additives and pharmaceutical ingredients, on vesicle membranes and, consequently, on their stability. Pluronic-modified liposomes were then loaded with curcumin. It was observed that particle sizes and polydispersity of vesicles were significantly decreased after adding the diverse pluronics, whereas the in vitro release of curcumin was slower compared to the release from conventional liposomes. Pluronics were also able to enhance thermal and pH stability of vesicles, in addition to significantly increase the absorption of curcumin in in vitro simulated gastrointestinal tract studies (Lia et al. 2018 ).
Untargeted liposomes loaded with anticancer drugs are the most successful nano-drug delivery systems translated into clinical applications, including the treatment of hematological cancers. Nanoliposomes for the simultaneous delivery of berberine chloride, antineoplastic drug, and tariquidar, P-gp efflux pump modulator, were formulated to enhance berberine chloride intracellular concentration in doxorubicin-resistant human erythroleukemia cells (K562/DOXO) due to Pg-p overexpression and to contemporaneously reduce tariquidar toxicity. Developed nanoliposomes had sizes around 128 nm and good PdI ( ca. 0.20) and ζ-potential ( ca. -20 mV). The stability of nanoliposomes in the cell culture medium showed only a slight variance in average sizes, PdI and encapsulation efficiency of berberine chloride and tariquidar. Analysis by transmission electron microscopy also evidenced the ability of developed nanoliposomes to enter in both cell lines by receptor-mediated endocytosis, with significant increase in berberine chloride uptake by K562/DOXO cell line. Moreover, formulated tariquidar had less toxic effects (lower cell death by necrosis) than free molecule, as nanoliposomes controlled tariquidar release, with a potential benefit in clinical therapy (Vanti et al. 2021b ).
Nanoliposomes were also investigated to formulate the Serenoa repens L. (saw palmetto) carbon dioxide (CO 2 ) extract, complex mixture constituted of free fatty acids, sterols, phosphoglycerides, glycerides and carotenoids. The saw palmetto CO 2 extract has many biological properties, including anti-inflammation, anti-androgen and anti-proliferation activities, and it has been recently studied for the treatment of hair loss because of the inhibition of 5α-reductase enzyme, responsible of the conversion of testosterone to 5α-dihydrotestosterone. Nanoliposomes ( ca. 145 nm) loaded with 0.1% w/v of saw palmetto CO 2 extract were developed and fully characterized, in terms of Size, PdI, ζ-potential, morphology and encapsulation efficiency, for topical application (Vanti et al. 2021a ).
Some studies have also evidenced the possibility to load essential oils inside liposomes. Generally, essential oils are complex mixtures of volatile, liquid, odorous, flavor and strongly active compounds. Due to their various biological properties, principally antioxidant and antimicrobial, they have been widely used since the Middle Ages and currently they also may have several applications in different fields, from medicine and cosmetics to food. However, their high volatility and low stability to direct exposure to light, oxygen, heat, and humidity can limit their efficacy and potential use. Accordingly, nanocarriers which can definitely load the essential oils, represent an innovative challenge to optimise the essential oil formulation, overcoming these main limitations (Bilia et al. 2014a ; De Matos et al. 2019 ). The resulting nanocarriers are stable and efficient, easily and safely produced, capable of stabilizing the essential oil, modulating its release, optimising its activity and providing an innovative formulative approach to avoid irritancy in case of cutaneous administration (Bilia et al. 2019b ).
The essential oil of Artemisia annua L. was loaded in conventional liposomes and its anti-fungal activity was evaluated against 10 different drug-resistant Candida strains. Formulated liposomes, containing 10 mg/mL of essential oil, had sizes around 250 nm, whereas the encapsulation efficiency of artemisia ketone, the marker constituent of the essential oil, was ca. 75%. The minimum fungicidal concentration of Artemisia annua essential oil loaded in liposomes, ranging 5 to 10 mg/mL, was much inferior than that of free essential oil (Risaliti et al. 2020b ). Another study investigated the antioxidant, anti-inflammatory and antibacterial properties of Salvia triloba L. and Rosmarinus officinalis L. essential oils formulated in liposomes. The obtained systems, loaded with 100 mg/mL of Salvia triloba L. or Rosmarinus officinalis L. essential oil, presented encapsulation efficiency of camphor, the marker constituent, around 57% for Salvia triloba L. and around 65% for Rosmarinus officinalis L. Liposomes were physically stable over time, when stored at +4°C, and very active against Klebsiella pneumoniae (Risaliti et al. 2019 ). Specifically, liposomes enhanced the antibacterial activity of both essential oils. Their antioxidant and anti-inflammatory activities, evaluated by in vitro tests, were also increased in comparison to those of unformulated essential oils.
Pegylated liposomes
Surface functionalization of liposomes has played an important role in formulation of liposomes as pharmaceutical carries, to improve their pharmacokinetics and effectiveness. For instance, conventional liposomes, when administered intravenously, are recognized by opsonin, a serum-protein, and phagocyted in the reticuloendothelial system. In order to increase the circulation time of liposomes, the bilayer surface can be coated with a hydrophilic polymer, such as the polyethylene glycol, increasing repulsive forces between liposomes and serum-components . Such surface modified liposomes have been defined as pegylated or stealth liposomes (Riaz et al. 2018 ).
Therefore, in a further study, pegylated liposomes, loaded with salvianolic acid B, were developed and tested in the same animal model of neuropathic pain reported for verbascoside (Isacchi et al. 2016 ). Salvianolic acid B was effective against mechanical hyperalgesia 15 min after administration, when injected intraperitoneally at a dose of 100 mg/kg. The antihyperalgesic activity prolonged for 30 min after administration, and the effect was still significant after 45 min (Isacchi et al. 2011b ).
Some studies report instead on the performance of conventional and long circulating liposomes (pegylated) loaded with artemisinin, a sesquiterpene lactone isolated from the Artemisia annua L., well known as very active antimalarial drug. Conventional and long circulating liposomes, with sizes of 130–140 nm and encapsulation efficacy higher than 70%, were injected in healthy mice. Free artemisinin was quickly cleared from plasma and it was almost not more detectable 1 hour after the injection. By contrast, artemisinin was still detectable after 3 and 24 hours when formulated in conventional or pegylated liposomes, respectively. The area under the curve (0-24 h) was six times increased with respect to free artemisinin. In particular, artemisinin half-life was enhanced more than 5-fold with long circulating liposomes (Isacchi et al. 2011a ). The same liposomes were tested in Plasmodium berghei NK-65 infected mice, a successful malaria model, at the dose of 50 mg/kg/day for artemisinin and 100 mg/kg/day for artemisinin plus curcumin. In mice treated with artemisinin-loaded liposomes or artemisinin plus curcumin-loaded liposomes (conventional and pegylated) was observed an immediate antimalarian effect. Particularly, artemisinin-loaded long circulating liposomes showed the most conspicuous and statistically significant activity (Isacchi et al. 2012 ). As artemisinin attracted increasing interest over years also for its antitumor properties in a variety of cancer cells, in a further study it was loaded in long circulating liposomes decorated with transferrin, in order to increase the up-take in tumoral cells. Specifically, cell uptake and cytotoxicity of liposomes were explored using the human colon carcinoma (HCT-8) cell line, because of the overexpression of transferrin receptors. An improved delivery of artemisinin was found for transferrin decorated liposomes with respect to the long-circulating vesicles, and enhanced cytotoxicity was also observed due to iron ions, obtaining a synergic anticancer effect (Leto et al. 2016 ).
Conventional and pegylated liposomes were also loaded with dihydroartemisinin, the natural metabolite of artemisinin and one of the most potent anticancer compounds, able to induce cancer cell death by apoptotic pathways. Cellular uptake efficiency of liposomes was determined by flow cytometry in MCF-7 cells. Higher internalization occurred for conventional liposomes rather than for stealth liposomes, probably due to the hydrophilic steric barrier of polyethylene glycol molecules. Furthermore, cytotoxicity studies on these cancer cells evidenced an increased toxicity for the formulated dihydroartemisinin and absence of toxicity for blank formulations (Righeschi et al. 2014 ).
Stabilized nanovesicles
Liposomes mostly consist of phospholipids and cholesterol. However, some solvents (ethanol, glycerol, propylene glycol) can impart stability, deformability or elasticity to the bilayer membrane of liposomes, resulting in a considerable improved drug loading and permeability through biological barriers by several orders of magnitude (Carita et al. 2018 ; Fernández-García et al. 2019 ).
Glycerosomes are innovative liposomes for dermal and transdermal drug delivery, based on glycerol and characterized by high physical stability and excellent deformability (Manca et al. 2013 ). In a recent investigation Melissa officinalis L. essential oil was loaded inside glycerosomes containing 10% v/v of glycerol, and it was evaluated for its anti-herpetic activity against herpes simplex virus (HSV) type 1 (herpes labialis). The encapsulation efficiency of the essential oil in terms of citral and β-caryophyllene, major constituents, was found to be ca. 63% and 76%, respectively. Moreover, the developed glycerosomes loaded with Melissa officinalis L. essential oil showed extraordinary chemical and physical stability during 4 months of storage, and were very active in inhibiting HSV type 1 infection of mammalian cells in vitro , without producing cytotoxic effects (Vanti et al. 2020a ).
Glycerosomes and propylene glycol-nanovesicles loaded with essential oils of Origanum onites L. and Satureja thymbra L. were also investigated as safe and food-grade delivery systems. Essential oils represent in fact a valid alternative to synthetic preservatives in the food industry. However, in many cases their organoleptic impact in foodstuffs limits their usage, in addition to the high volatility and chemical instability which decrease their efficacy. Techniques such as the encapsulation in nanodelivery systems can address this problem. Both pure and formulated essential oils were evaluated against different food-borne pathogens and spoilage microorganisms. Propylene glycol‐nanovesicles loaded with Origanum onites L. essential oil were found to be the most active formulation against all tested strains. Additionally, in vitro studies on HaCaT cell line showed that nanovesicles loaded with the essential oils had no toxic effect. Overall, these studies unveiled that tested nanovesicles could represent potential biocontrol agents against fungal and bacterial food pathogens with promising GRAS status in mammalian systems, besides being an innovative and completely biodegradable approach for the prolonged and sustained release of the essential oils, preserving functional properties (Vanti et al. 2021c ).
Active nanovesicular carriers
Recently, innovative nanovesicular systems, escinosomes and ascosomes, were also investigated using natural bioactive molecules as vesicle bilayer components, and they were studied for the skin delivery of selected model drugs.
The first study was design to explore the conversion of the bioactive amphiphilic saponin escin, isolated from seeds of Aesculus hippocastanum L. and clinically used for its anti-inflammatory, anti-oedematous and venotonic properties, into vesicle bilayer forming component, because of the suited chemical structure and the fluidizing effect on phospholipid membranes. Escinosomes, the obtained nanovesicles made of phosphatidylcholine plus escin, retained escin inhibitory activity on hyaluronidase and were subsequently loaded with berberine chloride, salt of the natural quaternary isoquinoline alkaloid berberine isolated from several medicinal plants. Berberine chloride was selected as model drug of low skin absorption. Empty and berberine chloride-loaded escinosomes displayed optimal characteristics for skin delivery, with high deformability, optimal physical stability, good encapsulation efficiency of berberine chloride (about 67%) and proper release rate of drug (about 75% after 24 h), (Vanti et al. 2019 ). Subsequently, escinosomes were gelled using hydroxypropyl methylcellulose. Developed escinosome-hydrogels combined the benefits of a controlled release and improved transdermal permeability of both escin and berberine chloride, thanks to the escinosome components, with optimised viscosity properties thanks to the polysaccharide matrix. The escinosome-hydrogels also showed a very good safety profile with no potential skin irritation, evaluated by in vivo acute dermal irritation/corrosion test on Sprague-Dawley rats (Vanti et al. 2020b ).
Ascosomes represent a further innovative nanocarrier for cutaneous application made of phosphatidylcholine plus derivates of L-ascorbyl acid, ascorbyl octanoate or ascorbyl decanoate, investigated as bioactive constituents of the vesicle bilayer. L-ascorbic acid (vitamin C) plays in fact an important role in the prevention and treatment of a large number of chronic diseases, including skin disorders, but it hardly penetrate the stratum corneum. The synthesis of amphiphilic derivates is a promising approach to enhance its skin penetration. In addition, because of their amphiphilic nature, ascorbyl derivates form supramolecular assemblies in aqueous dispersions, and they have been investigated as potential constituents for drug delivery systems. Obtained nanovesicles, ascosomes, were thus investigated as potential nanocarriers for the skin delivery of khellin, a natural furanochromone with various applications in skin pathologies and selected as model lipophilic drug. Developed ascosomes increased the cutaneous absorption of khellin and retained the antioxidant properties of ascorbic acid (Risaliti et al. 2020a ). Subsequently, a hydrogel of khellin-loaded ascosomes was developed using hydroxyethyl cellulose, in order to overcome the short residence time of the liquid formulation upon application on the skin, and to enhance the stability of the vesicles preventing their aggregation. The hydrogel of khellin-loaded ascosomes demonstrated a good safety profile in rats by the acute dermal irritation/corrosion test. Liver and dermal histological and pathological analyses also indicated that khellin formulated in the ascosome-hydrogel had no toxic effects (Risaliti et al. 2021 ).
Perspectives of lipid vesicles
The development of vesicles as carriers for therapeutic molecules is an ever-growing research area. Conventional liposomes, stabilized nanovesicles (glycerosomes and propylene glycol-nanovesicles), active nanovesicular carriers (escinosomes and ascosomes) have shown excellent potential in (co-)delivering natural products which belong to different chemical classes, namely phenylpropanoids (verbascoside, curcumin), flavonoids (naringenin), sesquiterpene lactones (artemisinin), saponins (escin), alkaloids (berberine chloride), furanochromones (khellin), or carbon dioxide extracts ( Serenoa repens L.) and essential oils ( Artemisia annua L., Salvia triloba L., Rosmarinus officinalis L., Melissa officinalis L., Origanum onites L., Satureja thymbra L.). All these vesicular systems, besides being biocompatible and suitable for all the administration routes, demonstrated to improve the biopharmaceutical properties of loaded natural drugs or to increase their chemical stability, with the aim of reaching an effective pharmacological activity. Lipid vesicles are still the most widely studied colloidal drug delivery systems, which also find great application in the clinical practice.
Nanocochleates
Nanocochleates represent an alternative platform to vesicles in order to overcome their main limitations, such as possible drug leakage, due to release processes, and low stability of the colloid in biological fluids. Cochleates were firstly observed when phosphatidylserine liposomes were treated with divalent metal cations, mainly magnesium and calcium. They are characterized by an exceptional stability due to their cylindrical shape and distinctive multi-layered structure (Fig. 2 ) (Bozó et al. 2017 ; Tilawat & Bonde 2021 ) and they were studied to formulate different kind of natural products.
Thymus vulgaris L. essential oil was loaded in nanocochleates (1 mg/mL), obtaining an encapsulation efficiency of the major constituents, thymol and carvacrol, of about 46% and 51%, respectively. Developed nanocochleates, ranging from ca. 210 to 250 nm, preserved the strong antioxidant activity of the unformulated Thymus vulgaris essential oil and were found to be suitable to provide a practical dosage form (Asprea et al. 2017 ). Nanocochleates have been also investigated as delivery systems of andrographolide, extensively studied for its wide spectrum of biological activities and selected as model drug because of its low water solubility and gastrointestinal instability. The developed andrographolide-loaded nanocochleates were found to be stable after lyophilisation and resuspension in distilled water, as well as after incubation in simulated gastric and intestinal media. They also demonstrated an elevated safety profile both in macrophages and 3T3 fibroblasts even at high concentrations, in addition to extraordinary uptake properties in macrophages, evaluated by a fluorescent probe (Asprea et al. 2019 ).
Nanocochleate is an extremely biocompatible system with excellent stability due to the unique compact structure, gaining increasing interest in pharmaceutical research as nanocarrier.
There is an increasing interest for nano-drug delivery systems for their potential application in clinic, both for local and systemic administration of drugs. Concurrently, natural products, which include a large and diverse group of substances from various natural sources, have assumed an exceptional importance for the prevention and cure of many diseases. This is due to their unique structure and their pleiotropic effects by targeting and modulating multiple pathways. For these reasons, natural products could represent the ideal drugs to a realistic approach of many diseases, especially those with emerging resistance to monofunctional agents, and they are suitable approaches against multifactorial and complex diseases, especially cancer and diabetes. By contrast, natural products suffer of many limitations and the majority of constituents are not “drug like”. Accordingly, the various nanovectors have been attempted as potential delivery systems for natural products, increasing the stability and solubility of loaded drugs and, thus, making them suitable for the administration. These nanostructures are also able to improve the biodistribution, with a consequent increased efficacy, as well as to favour the accumulation at target sites, reducing the adverse effects. Furthermore, the selection of biocompatible nanomaterials allow to decrease the formulation toxicity.
Ahuja R, Panwar N, Meena J, Singh M, Sarkar DP, Panda AK (2020) Natural products and polymeric nanocarriers for cancer treatment: a review. Environ Chem Lett. https://doi.org/10.1007/s10311-020-01056-z
Article Google Scholar
Asprea M, Leto I, Bergonzi MC, Bilia AR (2017) Thyme essential oil loaded in nanocochleates: encapsulation efficiency, in vitro release study and antioxidant activity. LWT 77:497–502
Article CAS Google Scholar
Asprea M, Tatini F, Piazzini V, Rossi F, Bergonzi MC, Bilia AR (2019) Stable, Monodisperse, and Highly Cell-Permeating Nanocochleates from Natural Soy Lecithin Liposomes. Pharmaceutics 11(1). pii: E34. https://doi.org/10.3390/pharmaceutics11010034
Barradas TN, Silva KGDH (2020) Nanoemulsions of essential oils to improve solubility, stability and permeability: a review. Environ Chem Lett. https://doi.org/10.1007/s10311-020-01142-2
Bergonzi MC, Hamdouch R, Mazzacuva F, Isacchi B, Bilia AR (2014) Optimization, characterization and in vitro evaluation of curcumin microemulsions. LWT-Food Sci Technol 59:148–155
Bergonzi MC, Guccione C, Grossi C, Piazzini V, Torracchi A, Luccarini I, Casamenti F, Bilia AR (2016) Albumin nanoparticles for brain delivery: a comparison of chemical versus thermal methods and in vivo behaviour. ChemMedChem 11(16):1840–1849. https://doi.org/10.1002/cmdc.201600080
Bilia AR, Guccione C, Isacchi B, Righeschi C, Firenzuoli F, Bergonzi MC (2014a) Essential oils loaded in nanosystems: a developing strategy for a successful therapeutic approach. Evid Based Complement Alternat Med. https://doi.org/10.1155/2014/651593
Bilia AR, Isacchi B, Righeschi C, Guccione C, Bergonzi MC (2014b) Flavonoids loaded in nanocarriers: an opportunity to increase oral bioavailability and bioefficacy. Food Nutr Sciences 5:1212–1227
Bilia AR, Piazzini V, Guccione C, Risaliti L, Asprea M, Capecchi G, Bergonzi MC (2017) Improving on nature: the role of nanomedicine in the development of clinical natural drugs. Planta Med 83:366–381
Bilia AR, Piazzini V, Asprea M, Risaliti L, Vanti G, Bergonzi MC (2018) Plants extracts loaded in nanocarriers: an emergent formulating approach. NPC 13:1157–1160
Google Scholar
Bilia AR, Nardiello P, Piazzini V, Leri M, Bergonzi MC, Bucciantini M, Casamenti F (2019a) Successful brain delivery of andrographolide loaded in human albumin nanoparticles to TgCRND8 mice, an Alzheimer’s disease mouse model. Front Pharmacol. https://doi.org/10.3389/fphar.2019.00910
Bilia AR, Piazzini V, Risaliti L, Vanti G, Casamonti M, Wang M, Bergonzi MC (2019b) Nanocarriers: a successful tool to increase solubility, stability and optimise bioefficacy of natural constituents. Curr Med Chem 25:1–24
Bilia AR, Piazzini V, Bergonzi MC (2020) Nanotechnology Applications for Natural Products Delivery. Sustainable Agriculture Reviews 44. 1–46. Springer, Cham
Bonaccini L, Karioti A, Bergonzi MC, Bilia AR (2015) Effects of Salvia miltiorrhiza on CNS neuronal injury and degeneration: a plausible complementary role of tanshinones and depsides. Planta Med 81:1003–1016
Bozó T, Wacha A, Mihály J, Bóta A, Kellermayer MSZ (2017) Dispersion and stabilization of cochleate nanoparticles. Eur J Pharm Biopharm 117:270–275
Bozzuto G, Molinari A (2015) Liposomes as nanomedical devices. Int J Nanomedicine 10:975–999
Cameron, A., Ewen, M., Auton, M., & Abegunde, D. (2011). The world medicines situation 2011. Medicines prices, availability and affordability,3
Carita AC, Eloy JO, Chorilli M, Lee RJ, Leonardi GR (2018) Recent advances and perspectives in liposomes for cutaneous drug delivery. Curr Med Chem 25(5):606–635
Casamonti M, Risaliti L, Vanti G, Piazzini V, Bergonzi MC, Bilia AR (2019) Andrographolide loaded in micro- and nano-formulations: improved bioavailability, target-tissue distribution, and efficacy of the “king of bitters.” Engineering 5:69–75
Cecchi L, Piazzini V, D’Ambrosio M, Luceri C, Rocco F, Innocenti M, Vanti G, Mulinacci N, Bergonzi MC (2020) Formulation of a Phenol-Rich Extract from Unripe Olives (Olea europaea L.) in Microemulsion to Improve Its Solubility and Intestinal Permeability. Molecules, 25(14), 3198. https://doi.org/10.3390/molecules25143198
Chamundeeswari M, Jeslin J, Verma ML (2019) Nanocarriers for drug delivery applications. Environ Chem Lett 17(2):849–865
Chaudhari VS, Murty US, Banerjee S (2020) Lipidic nanomaterials to deliver natural compounds against cancer: a review. Environ Chem Lett 18:1803–1812
Chaudhary S, Garg T, Murthy R et al (2015) Development, optimization and evaluation of long chain nanolipid carrier for hepatic delivery of silymarin through lymphatic transport pathway. Int J Pharm 485:108–121
Chen-Yu G, Chun-Fen Y, Qi-Lu L, Qi T, Yan-wei X, Wei-na L, Guang-xi Z (2012) Development of a quercetin-loaded nanostructured lipid carrier formulation for topical delivery. Int J Pharm 430:292–298
Chis AA, Dobrea C, Morgovan C, Arseniu AM, Rus LL, Butuca A, Juncan AM, Totan M, Vonica-Tincu AL, Cormos G, Muntean AC, Muresan ML, Gligor FG, Frum A (2020) Applications and limitations of dendrimers in biomedicine. Molecules (basel, Switzerland) 25(17):3982
Das S, Chaudhury A (2010) Recent advances in lipid nanoparticle formulations with solid matrix for oral drug delivery. AAPS PharmSciTech 12:62–76
De Matos SP, Teixeira HF, de Lima ÁA, Veiga-Junior VF, Koester LS (2019) Essential oils and isolated terpenes in nanosystems designed for topical administration: a review. Biomolecules 9(4):138. https://doi.org/10.3390/biom9040138
Divya K, Jisha MS (2018) Chitosan nanoparticles preparation and applications. Environ Chem Lett 16(1):101–112
Dragicevic, N., & Maibach, H. I. (Eds.). (2017). Percutaneous penetration enhancers drug penetration into/through the skin: Methodology and general considerations. Springer
Elzoghby AO, Samy WM, Elgindy NA (2012) Albumin-based nanoparticles as potential controlled release drug delivery systems. J Controll Rele: Offic J Controll Rele Soc 157(2):168–182
Falconieri MC, Adamo M, Monasterolo C, Bergonzi MC, Coronnello M, Bilia AR (2017) New dendrimer-based nanoparticles enhance curcumin solubility. Planta Med 83(5):420–425
CAS Google Scholar
Fang JY, Leu YL (2006) Prodrug strategy for enhancing drug delivery via skin. Curr Drug Discov Technol 3(3):211–224
Fernández-García R, Lalatsa A, Statts L, Bolás-Fernández F, Ballesteros MP, Serrano DR (2020) Transferosomes as nanocarriers for drugs across the skin: quality by design from lab to industrial scale. Int J Pharm 573:118817
Ghiasia Z, Esmaelia F, Aghajania M, Ghazi-Khansarib M, Faramarzic MA, Aman A (2019) Enhancing analgesic and anti-inflammatory effects of capsaicin when loaded into olive oil nanoemulsion: an in vivo study. Int J Pharm 559:341–347
Gillet A, Lecomte F, Hubert P, Ducat E, Evrard B, Piel G (2011) Skin penetration behaviour of liposomes as a function of their composition. Eur J Pharm Biopharm 79(1):43–53
Graverini G, Piazzini V, Landucci E, Pantano D, Nardiello P, Casamenti F, Pellegrini-Giampietro DE, Bilia AR, Bergonzi MC (2018) Solid lipid nanoparticles for delivery of andrographolide across the blood-brain barrier: in vitro and in vivo evaluation. Colloids Surf B Biointerfaces 161:302–313
Grossi C, Guccione C, Isacchi B, Bergonzi MC, Luccarini I, Casamenti F, Bilia AR (2017) Development of blood-brain barrier permeable nanoparticles as potential carriers for salvianolic acid B to CNS. Planta Med 83(5):382–391
Guccione C, Oufir M, Piazzini V, Eigenmann DE, Jähne EA, Zabela V, Faleschini MT, Bergonzi MC, Smiesko M, Hamburger M, Bilia AR (2017) Andrographolide-loaded nanoparticles for brain delivery: Formulation, characterisation and in vitro permeability using hCMEC/D3 cell line. Eur J Pharm Biopharm 119:253–263
Guccione C, Bergonzi MC, Awada KM, Piazzini V, Bilia AR (2018) Lipid nanocarriers for oral delivery of Serenoa repens CO2 extract: a study of microemulsion and self-microemulsifying drug delivery systems. Planta Med 84:736–742
Gupta S, Bansal R, Ali J, Gabrani R, Dang S (2014) Development and characterization of Polyphenon 60 and caffeine microemulsion for enhanced antibacterial activity. BioMed Res Int, Article ID 932017, 7 pages. https://doi.org/10.1155/2014/932017
Han L, Fu Y, Cole AJ, Liu J, Wang J (2012) Co-encapsulation and sustained-release of four components in ginkgo terpenes from injectable PELGE nanoparticles. Fitoterapia 83:721–731
Iqbal B, Ali J, Baboota S (2018) Recent advances and development in epidermal and dermal drug deposition enhancement technology. Int J Dermatol 57(6):646–660
Isacchi B, Arrigucci S, la Marca G, Bergonzi MC, Vannucchi MG, Novelli A, Bilia AR (2011a) Conventional and long-circulating liposomes of artemisinin: preparation, characterization, and pharmacokinetic profile in mice. J Liposome Res 21:237–244
Isacchi B, Fabbri V, Galeotti N, Bergonzi MC, Karioti A, Ghelardini C, Vannucchi MG, Bilia AR (2011b) Salvianolic acid B and its liposomal formulations: anti-hyperalgesic activity in the treatment of neuropathic pain. Eur J Pharm Sciences 44:552–558
Isacchi B, Bergonzi MC, Grazioso M, Righeschi C, Pietretti A, Severini C, Bilia AR (2012) Artemisinin and artemisinin plus curcumin liposomal formulations: enhanced antimalarial efficacy against Plasmodium bergheiinfected mice. Eur J Pharm Biopharm 80:528–534
Isacchi B, Bergonzi MC, Iacopi R, Ghelardini C, Galeotti N, Bilia AR (2016) Liposomal formulation to increase stability and prolong antineuropathic activity of verbascoside. Planta Med 83:412–419
Ita KB (2016) Prodrugs for transdermal drug delivery–trends and challenges. J Drug Target 24(8):671–678
Jain H, Chella N (2020) Methods to improve the solubility of therapeutical natural products: a review. Environ Chem Lett. https://doi.org/10.1007/s10311-020-01082-x
Kang BK, Lee JS, Chon SK, Jeong SY, Yuk SH, Khang G, Lee HB, Cho SH (2004) Development of self-microemulsifying drug delivery systems (SMEDDS) for oral bioavailability enhancement of simvastatin in beagle dogs. Int J Pharm 274:65–73
Kesarwani K, Gupta R (2013) Bioavailability enhancers of herbal origin: an overview. Asian Pac J Trop Biomed 3(4):253–266
Khan IA, Smillie T (2012) Implementing a “quality by design” approach to assure the safety and integrity of botanical dietary supplements. J Nat Prod 75(9):1665–1673
Kumar DL, Sarkar P (2018) Encapsulation of bioactive compounds using nanoemulsions. Environ Chem Lett 16(1):59–70
Kumar R, Jha D, Panda AK (2019) Antimicrobial therapeutics delivery systems based on biodegradable polylactide/polylactide-co-glycolide particles. Environ Chem Lett 17(3):1237–1249
Leto I, Coronnello M, Righeschi C, Bergonzi MC, Mini E, Bilia AR (2016) Enhanced efficacy of artemisinin loaded in transferrin-conjugated liposomes versus stealth liposomes against HCT-8 colon cancer cells. ChemMedChem 11(16):1745–1751
Lia Z-L, Penga S-F, Chena X, Zhua Y-Q, Zoua L-Q, Liua W, Liu C-M (2018) Pluronics modified liposomes for curcumin encapsulation: Sustained release, stability and bioaccessibility. Food Res Intern 108:246–253
Manca ML, Zaru M, Manconi M, Lai F, Valenti D, Sinico C, Fadda AM (2013) Glycerosomes: a new tool for effective dermal and transdermal drug delivery. Int J Pharm 455:66–74
McClements DJ (2012) Nanoemulsions versus Microemulsions: Terminology, Differences, and Similarities. Soft Matter 8:1719–1729
Mehnert W, Mäder K (2012) Solid lipid nanoparticles production, characterization and applications. Adv Drug Deliv Rev 64:83–101
Mehta P, Shah R, Lohidasan S, Mahadik KR (2015) Pharmacokinetic profile of phytoconstituent (s) isolated from medicinal plants—a comprehensive review. J Tradit Complement Med 5(4):207–227
Mishra V, Bansal KK, Verma A, Yadav N, Thakur S, Sudhakar K, Rosenholm JM (2018) Solid lipid nanoparticles: emerging colloidal nano drug delivery systems. Pharmaceutics 10(4):191
Mu H, Holm R (2018) Solid lipid nanocarriers in drug delivery: characterization and design. Expert Opin Drug Deliv 15(8):771–785 (PMID: 30064267)
Newman DJ, Cragg GM (2020) Natural products as sources of new drugs over the nearly four decades from 01/1981 to 09/2019. J Nat Prod 83(3):770–803. https://doi.org/10.1021/acs.jnatprod.9b01285
Parhi R (2020) Drug delivery applications of chitin and chitosan: a review. Environ Chem Lett 18(3):577–594
Paroha S, Dewangan RP, Dubey RD, Sahoo PK (2020) Conventional and nanomaterial-based techniques to increase the bioavailability of therapeutic natural products: a review. Environ Chem Lett 18:1767–1778
Piazzini V, Monteforte E, Luceri C, Bigagli E, Bilia AR, Bergonzi MC (2017a) Nanoemulsion for improving solubility and permeability of Vitex agnus-castus extract: formulation and in vitro evaluation using PAMPA and Caco-2 approaches. Drug Deliv 24:380–390
Piazzini V, Rosseti C, Bigagli E, Luceri C, Bilia AR, Bergonzi MC (2017b) Prediction of permeation and cellular transport of Silybum marianum extract formulated in a nanoemulsion by using PAMPA and Caco-2 Cell models. Planta Med 83:1184–1193
Piazzini V, Bigagli E, Luceri C, Bilia AR, Bergonzi MC (2018a) Enhanced solubility and permeability of salicis cortex extract by formulating as a microemulsion. Planta Med 84:976–984
Piazzini V, Lemmi B, D’Ambrosio M, Cinci L, Luceri C, Bilia AR, Bergonzi MC (2018b) Nanostructured lipid carriers as promising delivery systems for plant extracts: the case of silymarin. Appl Sci 8:1163
Piazzini V, D'Ambrosio M, Luceri C, Cinci L, Landucci E, Bilia AR, Bergonzi MC (2019) Formulation of nanomicelles to improve the solubility and the oral absorption of silymarin. Molecules 24(9). pii: E1688
Poonia N, Kharb R, Lather V, Pandita D (2016) Nanostructured lipid carriers: versatile oral delivery vehicle. Future Sci OA 2:FSO135
Riaz MK, Riaz MA, Zhang X, Lin C, Wong KH, Chen X, Yang Z (2018) Surface functionalization and targeting strategies of liposomes in solid tumor therapy: a review. Int J Mol Sci 19(1):195
Righeschi C, Coronnello M, Mastrantoni A, Isacchi B, Bergonzi MC, Mini E, Bilia AR (2014) Strategy to provide a useful solution to effective delivery of dihydroartemisinin: development, characterization and in vitro studies of liposomal formulations. Colloids Surf B Biointerfaces 116:121–127
Righeschi C, Bergonzi MC, Isacchi B, Bazzicalupi C, Gratteri P, Bilia AR (2016) Enhanced curcumin permeability by SLN formulation: The PAMPA approach. LWT - Food Sci Technol 66:475–483
Risaliti L, Kehagia A, Daoultzi E, Lazari D, Bergonzi MC, Vergkizi-Nikolakaki S, Hadjipavlou-Litina D, Bilia AR (2019) Liposomes loaded with salvia triloba and Rosmarinus officinalis essential oils: In vitro assessment of antioxidant, antiinflammatory and antibacterial activities. J Drug Del Sci Technol 51:493–498
Risaliti L, Ambrosi M, Calamante M, Bergonzi MC, Nostro PL, Bilia AR (2020a) Preparation and characterization of ascosome vesicles loaded with khellin. J Pharm Sci 109(10):3114–3124
Risaliti L, Pini G, Ascrizzi R, Donato R, Sacco C, Bergonzi MC, Salvatici MC, Bilia AR (2020b) Artemisia annua essential oil extraction, characterization, and incorporation in nanoliposomes, smart drug delivery systems against Candida species. J Drug Deliv Sci Technol 59:101849
Risaliti L, Yu X, Vanti G, Bergonzi MC, Wang M, Bilia AR (2021) Hydroxyethyl cellulose hydrogel for skin delivery of khellin loaded in ascosomes: characterization, in vitro/in vivo performance and acute toxicity. Int J Biol Macromol 179:217–229
Rodrigues T, Reker D, Schneider P, & Schneider G. (2016). Counting on natural products for drug design. Nature chemistry, 8(6), 531
Saini A, Panwar D, Panesar PS, Bera MB (2020) Encapsulation of functional ingredients in lipidic nanocarriers and antimicrobial applications: a review. Environ Chem Lett. https://doi.org/10.1007/s10311-020-01109-3
Saka R, Chella N. Nanotechnology for delivery of natural therapeutic substances: a review (2020). Environ Chem Lett. https://doi.org/10.1007/s10311-020-01103-9
Scioli Montoto S, Muraca G, Ruiz ME (2020) Solid Lipid nanoparticles for drug delivery: pharmacological and biopharmaceutical aspects. Front Mol Biosci 7:319
Sherje AP, Jadhav M, Dravyakar BR, Kadam D (2018) Dendrimers: a versatile nanocarrier for drug delivery and targeting. Int J Pharm 548(1):707–720
Sithole MN, Choonara YE, du Toit LC, Kumar P & Pillay V (2017). A review of semi-synthetic biopolymer complexes: modified polysaccharide nano-carriers for enhancement of oral drug bioavailability. Pharm. Dev. Technol, 22(2), 283–295
Tahmasebi Mirgani M, Isacchi B, Sadeghizadeh M, Marra F, Bilia AR, Mowla SJ, Najafi F, Babaei E (2014) Dendrosomal curcumin nanoformulation downregulates pluripotency genes via miR-145 activation in U87MG glioblastoma cells Int J Nanomedicine. 9:403–417
Tamjidi F, Shahedi M, Varshosaz J, Nasirpour A (2013) Nanostructured lipid carriers (NLC): a potential delivery system for bioactive food molecules. Innov Food Sci Emerg Technol 19:29–43
Tartaro G, Mateos H, Schirone D, Angelico R, Palazzo G (2020) Microemulsion microstructure(s): a tutorial review. Nanomaterials (basel, Switzerland) 10(9):1657. https://doi.org/10.3390/nano10091657
Tayeb HH, Sainsbury F (2018) Nanoemulsions in drug delivery: formulation to medical application. Nanomedicine (lond) 13(19):2507–2525
Tilawat M, Bonde S (2021) Nanocochleates: A potential drug delivery system. J Mol Liq 334:116115
Vanti G, Bani D, Salvatici MC, Bergonzi MC, Bilia AR (2019) Development and percutaneous permeation study of escinosomes, escin-based nanovesicles loaded with berberine chloride. Pharmaceutics 11(12):682. https://doi.org/10.3390/pharmaceutics11120682
Vanti G, Ntallis SG, Panagiotidis CA, Dourdouni V, Patsoura C, Bergonzi MC, Lazari D, Bilia, AR (2020a) Glycerosome of Melissa officinalis L. essential oil for effective anti-HSV Type 1. Molecules 25(14), 3111
Vanti G, Wang M, Bergonzi MC, Zhidong L, Bilia AR (2020b) Hydroxypropyl methylcellulose hydrogel of berberine chloride-loaded escinosomes: dermal absorption and biocompatibility. Int J Biol Macromol 164:232–241
Vanti G, Camilla Bergonzi M, Rita Bilia A (2021a) Development of nanoliposomes loaded with carbon dioxide serenoa repens (Saw Palmetto) extract. J Nanosci Nanotechnol 21(5):2943–2945
Vanti G, Coronnello M, Bani D, Mannini A, Bergonzi MC, Bilia AR (2021b) Co-Delivery of berberine chloride and tariquidar in nanoliposomes enhanced intracellular berberine chloride in a doxorubicin-resistant K562 cell line due to P-gp overexpression. Pharmaceutics 13(3):306. https://doi.org/10.3390/pharmaceutics13030306
Vanti G, Tomou EM, Stojković D, Ćirić A, Bilia AR, Skaltsa H (2021c) Nanovesicles loaded with origanum onites and satureja thymbra essential oils and their activity against food-borne pathogens and spoilage microorganisms. Molecules 26(8):2124. https://doi.org/10.3390/molecules26082124
Wan Y, Wang S, Firempong CK, Zhang H, Wang M, Zhang Y, Zhu Y, Yu J, Xu X (2017) Enhanced solubility and bioavailability of naringenin via liposomal nanoformulation: preparation and in vitro and in vivo evaluations. AAPS PharmSciTech 18:586–594. https://doi.org/10.1208/s12249-016-0537-8
Wang W, Zhu X, Li A, Xiao Y, Li K, Liu H, Cui D, Chen Y, Wang S (2012) Enhanced bioavailability and efficiency of curcumin for the treatment of asthma by its formulation in solid lipid nanoparticles. Int J Nanomed. https://doi.org/10.2147/ijn.s30428
Xie X, Tao Q, Zou Y, Zhang F, Guo M, Wang Y, Wang H, Zhou Q, Yu S (2011) PLGA nanoparticles improve the oral bioavailability of curcumin in rats: characterizations and mechanisms. J Agric Food Chem 59(17):9280–9289
Yang T, Sheng H-H, Feng N-P, Wei H, Wang ZT, Wang CH (2013) Preparation of andrographolide-loaded solid lipid nanoparticles and their in vitro and in vivo evaluations: characteristics, release, absorption, transports, pharmacokinetics, and antihyperlipidemic activity. J Pharm Sci 102:4414–4425
Zhang Y, Wang R, Wu J, Shen Q (2012) Characterization and evaluation of self-microemulsifying sustained-release pellet formulation of puerarin for oral delivery. Int J Pharm 427:337–344
Download references
Acknowledgements
The author thanks MIUR-Italy (“Progetto dipartimenti di eccellenza 2018-2022” allocated to Department of Chemistry “Ugo Schiff”, University of Florence, Italy).
Author information
Authors and affiliations.
Department of Chemistry “Ugo Schiff”, University of Florence, Via Ugo Schiff 6, 50019, Sesto Fiorentino, Florence, Italy
Giulia Vanti
You can also search for this author in PubMed Google Scholar
Corresponding author
Correspondence to Giulia Vanti .
Ethics declarations
Open access funding provided by Università degli Studi di Firenze within the CRUI-CARE Agreement.
Conflicts of interest
The author declares no conflict of interest
Additional information
Publisher's note.
Springer Nature remains neutral with regard to jurisdictional claims in published maps and institutional affiliations.
Rights and permissions
Open Access This article is licensed under a Creative Commons Attribution 4.0 International License, which permits use, sharing, adaptation, distribution and reproduction in any medium or format, as long as you give appropriate credit to the original author(s) and the source, provide a link to the Creative Commons licence, and indicate if changes were made. The images or other third party material in this article are included in the article's Creative Commons licence, unless indicated otherwise in a credit line to the material. If material is not included in the article's Creative Commons licence and your intended use is not permitted by statutory regulation or exceeds the permitted use, you will need to obtain permission directly from the copyright holder. To view a copy of this licence, visit http://creativecommons.org/licenses/by/4.0/ .
Reprints and permissions
About this article
Vanti, G. Recent strategies in nanodelivery systems for natural products: a review. Environ Chem Lett 19 , 4311–4326 (2021). https://doi.org/10.1007/s10311-021-01276-x
Download citation
Received : 10 December 2020
Accepted : 11 July 2021
Published : 28 July 2021
Issue Date : December 2021
DOI : https://doi.org/10.1007/s10311-021-01276-x
Share this article
Anyone you share the following link with will be able to read this content:
Sorry, a shareable link is not currently available for this article.
Provided by the Springer Nature SharedIt content-sharing initiative
- Natural products
- Nanovectors
- Delivery systems
- Polymeric and lipid nanosystems
- Bioavailability and efficacy
- Find a journal
- Publish with us
- Track your research
Natural products from the Lithistida: a review of the literature since 2000
Affiliation.
- 1 Harbor Branch Oceanographic Institution at Florida Atlantic University, Center for Marine Biomedical and Biotechnology Research, 5600 US 1 North, Fort Pierce, FL 34946, USA.
- PMID: 22363244
- PMCID: PMC3280575
- DOI: 10.3390/md9122643
Lithistid sponges are known to produce a diverse array of compounds ranging from polyketides, cyclic and linear peptides, alkaloids, pigments, lipids, and sterols. A majority of these structurally complex compounds have very potent and interesting biological activities. It has been a decade since a thorough review has been published that summarizes the literature on the natural products reported from this amazing sponge order. This review provides an update on the current taxonomic classification of the Lithistida, describes structures and biological activities of 131 new natural products, and discusses highlights from the total syntheses of 16 compounds from marine sponges of the Order Lithistida providing a compilation of the literature since the last review published in 2002.
Keywords: Lithistida; Theonella; desmas; lithistid; natural product.
Publication types
- Research Support, N.I.H., Extramural
- Research Support, Non-U.S. Gov't
- Biological Products / chemical synthesis
- Biological Products / chemistry
- Biological Products / isolation & purification*
- Biological Products / pharmacology
- HCT116 Cells
- Porifera / chemistry*
- Porifera / classification
- Time Factors
- Biological Products
Grants and funding
- R01 CA093455/CA/NCI NIH HHS/United States
- R01 CA093455-07/CA/NCI NIH HHS/United States
- R01-CA093455/CA/NCI NIH HHS/United States

An official website of the United States government
The .gov means it’s official. Federal government websites often end in .gov or .mil. Before sharing sensitive information, make sure you’re on a federal government site.
The site is secure. The https:// ensures that you are connecting to the official website and that any information you provide is encrypted and transmitted securely.
- Publications
- Account settings
Preview improvements coming to the PMC website in October 2024. Learn More or Try it out now .
- Advanced Search
- Journal List

The Traditional Medicine and Modern Medicine from Natural Products
Haidan yuan.
1 College of Pharmacy, Yanbian University, Yanji 133002, China; nc.ude.uby@nauydh (H.Y.); moc.361@8193naiqnaiq (Q.M.); nc.ude.uby@1260104102 (L.Y.)
2 Key Laboratory of Natural Resources of Changbai Mountain and Functional Molecules, Ministry of Education, Yanbian University, Yanji 133002, China
Qianqian Ma
Guangchun piao.
Natural products and traditional medicines are of great importance. Such forms of medicine as traditional Chinese medicine, Ayurveda, Kampo, traditional Korean medicine, and Unani have been practiced in some areas of the world and have blossomed into orderly-regulated systems of medicine. This study aims to review the literature on the relationship among natural products, traditional medicines, and modern medicine, and to explore the possible concepts and methodologies from natural products and traditional medicines to further develop drug discovery. The unique characteristics of theory, application, current role or status, and modern research of eight kinds of traditional medicine systems are summarized in this study. Although only a tiny fraction of the existing plant species have been scientifically researched for bioactivities since 1805, when the first pharmacologically-active compound morphine was isolated from opium, natural products and traditional medicines have already made fruitful contributions for modern medicine. When used to develop new drugs, natural products and traditional medicines have their incomparable advantages, such as abundant clinical experiences, and their unique diversity of chemical structures and biological activities.
1. Introduction
Since prehistoric times, humans have used natural products, such as plants, animals, microorganisms, and marine organisms, in medicines to alleviate and treat diseases. According to fossil records, the human use of plants as medicines may be traced back at least 60,000 years [ 1 , 2 ]. The use of natural products as medicines must, of course, have presented a tremendous challenge to early humans. It is highly probable that when seeking food, early humans often consumed poisonous plants, which led to vomiting, diarrhea, coma, or other toxic reactions—perhaps even death. However, in this way, early humans were able to develop knowledge about edible materials and natural medicines [ 3 ]. Subsequently, humans invented fire, learned how to make alcohol, developed religions, and made technological breakthroughs, and they learned how to develop new drugs.
Traditional medicines (TMs) make use of natural products and are of great importance. Such forms of medicine as traditional Chinese medicine (TCM), Ayurveda, Kampo, traditional Korean medicine (TKM), and Unani employ natural products and have been practiced all over the world for hundreds or even thousands of years, and they have blossomed into orderly-regulated systems of medicine. In their various forms, they may have certain defects, but they are still a valuable repository of human knowledge [ 2 , 4 ].
In the case of China, Western medicine was introduced in the sixteenth century, but it did not undergo any development until the nineteenth century. Before that, TCM was the dominant form of medical care in the country [ 5 ]. Now TCM still plays an important role in China, and it is constantly being developed. TCM is based on 5000 years of medical practice and experience, and is rich in data from “clinical experiments” which guarantee its effectiveness and efficacy. It has developed techniques with respect to such areas as correct dosage, methods of preparing and processing materials, and the appropriate time to collect the various medicinal parts of plants. It is notable that there is increasing convergence between TCM and modern medicine. With the development of modern technology, it has become possible to determine the pharmacology and mechanisms of action of many Chinese herbs, and TCM has become comprehensible in terms of modern medicine [ 6 , 7 , 8 , 9 ]. With advances in the theoretical background, therapeutic principles, associated technologies, and understanding of the life sciences, a clearer understanding of the active compounds of TCM has become possible [ 5 ].
At the beginning of the nineteenth century, the era of “modern” drugs began. In 1805, the first pharmacologically-active compound morphine was isolated by a young German pharmacist, Friedrich Sertürner, from the opium plant [ 10 , 11 ]. Subsequently, countless active compounds have been separated from natural products. Among them, some follow their traditional uses and the others do not. Later, the development of synthetic techniques led to a significant reduction in the importance of natural products, and there were concerns that the use of some natural products for medicinal purposes might be completely banned. However, natural products are important for the development of new drugs, and these products have been in constant use. Some type of medicines, such as anticancer, antihypertensive, and antimigraine medication, have benefited greatly from natural products [ 10 , 12 ].
The development of new drugs relying purely on modern technology appears to be reaching something of a limit. In developing new drugs, the pharmaceutical industry has tended to adopt high-throughput synthesis and combinatorial chemistry-based drug development since the 1980s; however, the considerable efforts made in this direction have not resulted in the expected drug productivity. Some large pharmaceutical companies are facing great challenges to develop new products. Over the past dozen years, increasing attention has accordingly been paid to natural products in the search for novel drugs in combination with new technology, such as high-throughput selection [ 13 , 14 ].
Natural products, which have evolved over millions of years, have a unique chemical diversity, which results in diversity in their biological activities and drug-like properties. Those products have become one of the most important resources for developing new lead compounds and scaffolds. Natural products will undergo continual use toward meeting the urgent need to develop effective drugs, and they will play a leading role in the discovery of drugs for treating human diseases, especially critical diseases [ 15 ].
2. Natural Products
Natural products have a wide range of diversity of multi-dimensional chemical structures; in the meantime, the utility of natural products as biological function modifiers has also won considerable attention. Subsequently, they have been successfully employed in the discovery of new drugs and have exerted a far-reaching impact on chemicobiology [ 16 , 17 , 18 ]. From the past century, the high structural diversity of natural products have been realized from the perspective of physical chemistry. Their efficacy is related to the complexity of their well-organized three-dimensional chemical and steric properties, which offer many advantages in terms of efficiency and selectivity of molecular targets. As a successful example of drug development from natural products, artemisinin and its analogs are presently in wide use for the anti-malaria treatment. This shows how research using natural products has made a significant contribution in drug development [ 19 , 20 ].
Among anticancer drugs approved in the time frame of about 1940–2002, approximately 54% were derived natural products or drugs inspired from knowledge related to such. For instance, the Vinca alkaloids from Catharanthus roseus , and the terpene paclitaxel from Taxus baccata , are among successful anticancer drugs originally derived from plants [ 12 , 21 ]. During the period between 1981 and 2002, the application of natural products in the development of new drugs—especially in the search for novel chemical structures—showed conspicuous success. In that 22-year time frame, drugs derived from natural products have been significant. That is especially true in the case of antihypertensives, where about 64% of newly-synthesized drugs have their origins in natural product structures [ 12 ].
Considering their incomparable chemical diversity and novel mechanisms of action, natural products have continued to play a pivotal role in many drug development and research programs. With time, those natural products have undergone interesting and meaningful developments in their ability to interact with numerous, varied biological targets, and some have become the most important drugs in health care system [ 14 , 22 , 23 ]. For example, plants, microorganisms, and animals manufacture small molecules, which have played a major role in drug discovery. Among 69 small-molecule new drugs approved from 2005 to 2007 worldwide, 13 were natural products or originated from natural products, which underlines the importance of such products in drug research and development [ 12 , 13 ].
Over the past 50 years, there has been a great diversity of new drugs developed using high-throughput screening methods and combinatorial chemistry; however, natural products and their derived compounds have continued to be highly-important components in pharmacopoeias. Of the reckoned 250,000–500,000 existing plant species, only a tiny proportion has been scientifically researched for bioactivities [ 13 ]. Therefore, there is great potential for future discoveries from plants and other natural products which, thus, offer huge potential in deriving useful information about novel chemical structures and their new types of action related to new drug development.
3. Traditional Medicines
TM is the oldest form of health care in the world and is used in the prevention, and treatment of physical and mental illnesses. Different societies historically developed various useful healing methods to combat a variety of health- and life-threatening diseases. TM is also variously known as complementary and alternative, or ethnic medicine, and it still plays a key role in many countries today [ 24 , 25 ].
The medicaments used in TM are mostly derived from natural products. In TM, “clinical trials” have been conducted since ancient times. In the case of TCM, considerable experience and advances have been accumulated and developed over the past thousands of years with respect to methods of preparation, selection of herbs, identification of medicinal materials, and the best time for obtaining various different plants. Appropriate processing and dose regulation are urgently needed in TCM to improve drug efficacy and reduce drug toxicity. Considerable amounts of data have been acquired through clinical experiments, and in this way TM has assisted in the development of modern drugs. Through its use of natural products, TM offers merits over other forms of medicine in such areas as the following: discovery of lead compounds and drug candidates; examining drug-like activity; and exploring physicochemical, biochemical, pharmacokinetic, and toxicological characteristics. If any form of TM is applied successfully, it may surprisingly assist in the development of new drugs, thereby resulting in many benefits, such as significant cost reductions.
TCM is now an inseparable part of the Chinese public health system. In recent years, TCM has gradually gained considerable approval as a complementary or alternative medicine in Western countries. Chinese herbal medicine, which is the most important component of TCM, is currently used in the health care of an estimated 1.5 billion people worldwide [ 26 , 27 ]. It should be noted that in TCM, several herbs and ingredients are combined according to strict rules to form prescriptions, which are referred to as formulas ( fang ji in Chinese). Commonly, a classic formula is composed of four elements—the “monarch”, “minister”, “assistant”, and “servant”—according to their different roles in the formula, each of which consists of one to several drugs. Ideally, these drugs constitute an organic group to produce the desired therapeutic effect and reduce adverse reactions [ 28 ].
Kampo is the TM of Japan. Between the fifth and sixth centuries, TCM was introduced to Japan from China; since then, TCM has been significantly altered and adapted by Japanese practitioners to meet their particular circumstances and gradually evolved into Kampo [ 29 ]. A recent study has found that some physicians in Japan use Kampo medicines in their daily practice—sometimes as the preferred medication [ 29 , 30 , 31 ]. Together with radiotherapy or chemotherapy, some Japanese physicians frequently utilize Kampo medicines in treating cancer patients. This indicates how modern Western medicine can be well integrated with TM [ 30 , 32 ]. As the use of Kampo continues to rise in conjunction with Western medicine, there is growing realization of the urgent need to study the interactions between these two types of medicines [ 28 ].
Unani is an ancient Greek holistic medical system with a history that can be traced back 2500 years [ 33 ]. Since the mid-1970s, when the WHO began to place a greater focus on TM, Unani has attracted considerable attention all over the world, especially in India, where it has been integrated into the national health care system [ 34 ].
It was reckoned by WHO that a large quantity of people in the world still depend on TMs for health care [ 35 ]. The current status of TM differs in different countries. In 2012, the total value of the TCM industry was equivalent to around one-third of the total for China’s pharmaceutical industry [ 36 ]. It has been determined that 80% of the population in Africa makes use of TM—either alone or in conjunction with conventional medicine [ 37 ]. By contrast, traditional Aboriginal medicine in Australia is in danger of vanishing owing to the prevalence of conventional medicine [ 38 ]. In the case of Israel with its ethnic diversity, modern medicine is prevailing, and TM is declining [ 39 ]. Many practitioners of Western medical science think such TM systems as being short of reliability; however, they are adopted by the majority of people in the world [ 35 ]. It is possible to produce remarkable synergy and yield great benefits in developing reformed medicines and new drugs by connecting powerful modern scientific techniques and methods with the reasonable ethnobotanical and ethnomedical experiences of TM. Characteristics of several TM systems are summarized in Table 1 .
Characteristics of several important traditional medicine systems.
4. Drugs Developed from Traditional Medicines that Follow the Traditional Uses
TM is too valuable to be ignored in the research and development of modern drugs. Though it has an enigmatic character, there are also wide contexts for its use in terms of non-Western medical technology or activities. In TM, a single herb or formula may contain many phytochemical constituents, such as alkaloids, terpenoids, flavonoids, etc. Generally speaking, these chemicals function alone or in conjunction with one another to produce the desired pharmacological effect [ 35 ]. It is notable that a lot of plant-originated drugs in clinical medicine today were derived from TM [ 21 ]. In addition, it has been demonstrated that the many valuable drugs derived from plants were discovered through their application in TM [ 2 ].
Almost 20 years ago, a thorough investigation of the pharmacopoeias of developed and developing nations and the associated world scientific literature was conducted as part of the WHO’s TM Program. The aim of that study was to determine whether TM really had inspired modern drug discoveries and whether there was any correlation between the current use of various compounds and their application in TM. The study focused on various compounds used in drugs derived from plants in different countries, and it established that TM had indeed played a significant role in developing effective new drugs. That study focused on 122 compounds, 80% of which were found to be related to pharmaceutical effects in folk medicine, and it was determined that these compounds originated from 94 plant species [ 2 ].
The acceptability, convenience, and accessibility of TMs have been, and will be, helpful for new drug research [ 13 ]. As noted above, artemisinin and other antimalarial drugs are examples of modern drugs based on TMs. Early in China’s Jin Dynasty, Doctor Hong Ge (AD 284–384) recorded the efficacy and related details of Artemisia annua L. in treating malaria in his book Zhou Hou Bei Ji Fang . That is the earliest record anywhere of treating malaria with Artemisia annua L., and it shows that Chinese physicians 1700 years ago had reached a sophisticated level of medical treatment [ 53 , 54 ].
Artemisinin is known as qinghaosu in Chinese, and its study has made significant progress, including the synthesis of new artemisinin analogs and derivatives, and research efforts into the biological activities and related mechanisms. As a result, artemisinin, as well as its effective derivatives, are extensively applied throughout the world as new-type anti-malarial drugs [ 55 ].
The discovery of artemisinin can be traced back to the 1960s, when tropical malaria was a serious problem during the Vietnam War. North Vietnam requested China to help tackle the malaria problem. The Chinese government approved a project for malaria control and drug research in 1967. The research group made its investigations and carried out a large-scale search of the literature on the subject. As part of the phytochemical and pharmacological research effort, a lot of Chinese herbal medicines were screened and investigated with respect to their toxicity or efficacy. Eventually artemisinin was derived from Artemisia annua L. in 1972 [ 53 , 55 , 56 ]. Artemisinin is quite different from previously-used antimalarial drugs, such as chloroquine, in that it has a novel structure, with a sesquiterpene lactone bearing a peroxy group, and it does not contain nitrogen heterocycles. Compared with previous antimalarial drugs, artemisinin has the merit of high efficiency, quick effect, and low toxicity. Artemisinin is effective in treating various forms of malaria, such as falciparum and cerebral malaria, which are resistant to chloroquine, and its mechanism of action is different from traditional antimalarial drugs. The discovery of artemisinin was a great success for TCM at a special period in China’s history, and it was achieved through a well-organized team of hundreds of researchers [ 56 ]. Since that breakthrough, scientists have conducted comprehensive research in such areas as pharmaceutical chemistry, organic synthetic chemistry, and chemical biology. Through etherification and esterification, they have produced a series of well-known new drugs, such as artemether and artesunate. Those drugs have improved efficacy and solubility, which are of benefit for patients receiving oral or intravenous administration and have overcome the high parasite recrudescence rate and low solubility of artemisinin [ 55 , 56 , 57 ]. Most importantly, one of these scientists, Youyou Tu, was just awarded the 2015 Nobel Medicine Prize for her significant devotion in discovering artemisinin.
The discovery of artemisinin illustrates how TCM constitutes a great store of knowledge about natural products, such as Chinese herbs, and holds much future promise. The discovery of successful new drugs can proceed by profiting from this knowledge [ 56 ]. Some drugs or compounds isolated from Chinese herbal medicines which follow the ethnomedical uses are summarized in Table 2 .
Some drugs or compounds isolated from Chinese herbal medicines which follow the traditional uses.
5. Drugs Developed from Natural Products
In clinical practice in China in the 1960s, it was found that Schisandra chinensis (Turcz.) Baill.—a traditional Chinese herb—had obvious enzyme-reducing and hepatoprotective effects. Chinese scientists then began isolating the chemical constituents of S. chinensis . In the subsequent total chemical synthesis and pharmacodynamic study of schisandrin C (which is one of the compounds of S. chinensis ), researchers found that the intermediate compound bifendate had a stronger pharmacological activity and that the cost of preparation was low. They discovered that it may be used to lower the enzyme content in the treatment of hepatitis B virus [ 57 ].
Since the end of the 1980s, chemists and pharmacologists at the Chinese Academy of Medical Sciences have been closely cooperating in studying the structure and activity relationships of bifendate and its analogs. As part of their research, a series of novel derivatives were synthesized. After screening using a number of chemical and pharmaceutical liver injury models, it was found that the hepatoprotective activities of the derivatives were closely related to the locations of dimethylenedioxy in two benzene rings, the length of the side-chain carboxylic acid, and the heterocycle between the two benzene rings. Finally, a new compound, bicyclol—formulated as 4,4″-dimethoxy-5,6,5′,6′-bis(methylene-dioxy)-2-hydroxy-methyl-2′-methoxycarbonyl biphenyl—was designed and synthesized. Bicyclol had greater in vivo absorption, and better bioavailability and biological activity, than bifendate owing to the introduction of the 6-hydroxymethyl group and 6′-carbomethoxy in the side chain [ 72 ]. Pharmacological results of bicyclol showed antifibrotic and hepatoprotective effects against liver injury and liver fibrosis induced by CCl 4 or other hepatotoxins in mice and rats; it also exhibited the antihepatitis virus effect in the 2.2.15 cell line and duck model with viral hepatitis [ 73 , 74 ].
In clinical trials, it was found that the increased levels of serum alanine aminotransferase and aspartate aminotransferase were dramatically decreased by bicyclol. It was also found that bicyclol prohibited hepatitis B virus replication in chronic hepatitis B patients [ 75 ]. Compared with previous anti-hepatitis drugs, bicyclol exhibited a more consolidated effect after the drug was discontinued; the rebound rate was low, with fewer adverse reactions and higher oral bioavailability [ 76 ]. Based on previous studies in such areas as synthesis, pharmacology, toxicology, pharmacokinetics, preparation, and quality control, researchers determined that the new antihepatitis drug bicyclol offered significant hepatoprotective effects, antihepatitis virus activity, and fewer adverse reactions [ 57 ]. Bicyclol has been approved for the treatment of chronic viral hepatitis in China since 2004 [ 73 ]. Bicyclol has independent intellectual property rights and belongs to Class 1 of China’s New Chemical Drug. The drug is one of the anti-inflammatory and hepatoprotective drugs recommended by the “Guidelines on Liver Disease Clinical Diagnosis and Treatment” in China, and it has been exported to many countries [ 57 , 76 ].
In the same decade in which Chinese scientists found that S. chinensis (Turcz.) Baill. had obvious enzyme-reducing and hepatoprotective effects, a program screening for cancer drugs from plants began in 1960 at the National Cancer Institute in the United States. Neither China nor the United States knew what the other was doing in this area. In that US project, 650 plant samples were gathered in three states. After the initial cytotoxicity tests were carried out using crude extracts, Taxus brevifolia was chosen for further research.
Taxol was isolated as a new compound from T. Brevifolia . Taxol has an unusual chemical structure and radically distinctive mechanism of action and was developed as a novel anticancer drug in subsequent decades. Nevertheless, the drug attracted little attention during the early stage of its development because of its poor solubility in water, low yield from natural products, and other disadvantages, particularly by the medical society. The story of Taxol involved many events that nearly resulted in discontinuation of the research. Fortunately, it underwent extraction, isolation, and structural determination; its activity against solid tumors and its mechanism of action were established, and it became developed for clinical practice. Finally, Taxol was approved by the US Food and Drug Administration for treating ovarian cancer in 1992—21 years after the initial breakthrough paper recording its isolation and structural identification. Taxol has remained a basic drug for treating various forms of cancer, and is still being used to develop new synergistic groups of anticancer drugs [ 77 , 78 , 79 ]. Some drugs or compounds isolated or developed from natural products are summarized in Table 3 .
Some drugs or compounds isolated or developed from natural products.
6. Discussion
Human history is also the history of medicines used to treat and prevent various diseases. To counter the danger from serious illnesses and to guarantee survival of the species, it is necessary to continually produce better drugs. With time, the use of these natural products as TM increased. Modern medicine has benefited considerably from TM in two areas: drugs with similar effects and drugs with different effects from those of TM. From the history of drug development, it is evident that many drugs have been derived as a result of inspiration from TM.
The application of, and research into, natural products are far from satisfactory. A number of problems need to be addressed in the future. For example, synergistic effects may exist among the compounds that occur in natural products; however, the modes and mechanisms of action are seldom very clear. It is, therefore, necessary to make full use of such synergetic effects toward improving the effectiveness of drugs. However, it is also requisite that any adverse effects of natural products be properly reduced to meet safety standards.
With the riches of modern technology, such as in synthesis, fermentation, pharmacology, pharmacodynamics—together with biological diversity, chemodiversity, and great breakthroughs in evolutionary techniques or concepts—combined with a wealth of knowledge about natural products, it will be possible to establish a large compound library for drug screening [ 89 ]. This will enhance the possibilities for individual treatment and prevention of disease. Humankind needs to learn more from natural products and traditional medicines.
In order to further promote the development of modern medical research on natural products, humans have to face up to various difficulties and challenges. Valuable information on natural products and TMs is mixed in a large number of documents, data, and useless rumors. Furthermore, one plant or formula of natural products and TMs contains a large number of chemical constituents, including active, invalid, and possible synergistic components. Therefore, great effort should be made at first to remove the dross and take the essence—precious experience of natural products and TMs. Furthermore, in many cases, the role of single compound from natural products and TMs is paid much attention to. However, as a matter of fact, one advantage of TM’s therapeutics is the “synergism”; that is, often multiple components in TMs play a synergistic role which is greater than that of the individual drug. In the meantime, the “1 disease, 1 target, 1 drug” mode cannot treat some complex diseases effectively, such as cardiovascular disease and diabetes. Thus, the treatment has seen a shift to the “multi-drugs and multi-targets” mode for combination therapies. Therefore, in the future, multidisciplinary collaborative research, closely cooperated with new ideas, such as network pharmacology and big data, will be possible to explain the synergism and other mechanisms of natural products and TMs from which more and better new drugs and treatment will be discovered and inspired.
Acknowledgments
This review was supported in part by research grants (No. 81260669 and 81560698) from National Natural Science Foundation of China, respectively.
Conflicts of Interest
The authors declare no conflict of interest.

Maintenance work is planned for Wednesday 1st May 2024 from 9:00am to 11:00am (BST).
During this time, the performance of our website may be affected - searches may run slowly and some pages may be temporarily unavailable. If this happens, please try refreshing your web browser or try waiting two to three minutes before trying again.
We apologise for any inconvenience this might cause and thank you for your patience.
Natural Product Reports
Marine natural products †.

* Corresponding authors
a School of Environment and Science, Griffith University, Gold Coast, Australia E-mail: [email protected]
b Griffith Institute for Drug Discovery, Griffith University, Brisbane, Australia
c School of Chemical Sciences, University of Auckland, Auckland, New Zealand
d Natural Products Branch, Developmental Therapeutics Program, Division of Cancer Treatment and Diagnosis, and Molecular Targets Program, Center for Cancer Research, National Cancer Institute, Frederick, MD, USA
e Centre for Biodiscovery, and School of Chemical and Physical Sciences, Victoria University of Wellington, Wellington, New Zealand
f School of Science, University of Waikato, Hamilton, New Zealand
Covering: January to the end of December 2022
This review covers the literature published in 2022 for marine natural products (MNPs), with 645 citations (633 for the period January to December 2022) referring to compounds isolated from marine microorganisms and phytoplankton, green, brown and red algae, sponges, cnidarians, bryozoans, molluscs, tunicates, echinoderms, the submerged parts of mangroves and other intertidal plants. The emphasis is on new compounds (1417 in 384 papers for 2022), together with the relevant biological activities, source organisms and country of origin. Pertinent reviews, biosynthetic studies, first syntheses, and syntheses that led to the revision of structures or stereochemistries, have been included. An analysis of NP structure class diversity in relation to biota source and biome is discussed.
Supplementary files
- Supplementary information PDF (11549K)
Article information

Download Citation
Permissions.

Marine natural products
A. R. Carroll, B. R. Copp, T. Grkovic, R. A. Keyzers and M. R. Prinsep, Nat. Prod. Rep. , 2024, 41 , 162 DOI: 10.1039/D3NP00061C
This article is licensed under a Creative Commons Attribution-NonCommercial 3.0 Unported Licence . You can use material from this article in other publications, without requesting further permission from the RSC, provided that the correct acknowledgement is given and it is not used for commercial purposes.
To request permission to reproduce material from this article in a commercial publication , please go to the Copyright Clearance Center request page .
If you are an author contributing to an RSC publication, you do not need to request permission provided correct acknowledgement is given.
If you are the author of this article, you do not need to request permission to reproduce figures and diagrams provided correct acknowledgement is given. If you want to reproduce the whole article in a third-party commercial publication (excluding your thesis/dissertation for which permission is not required) please go to the Copyright Clearance Center request page .
Read more about how to correctly acknowledge RSC content .
Social activity
Search articles by author, advertisements.
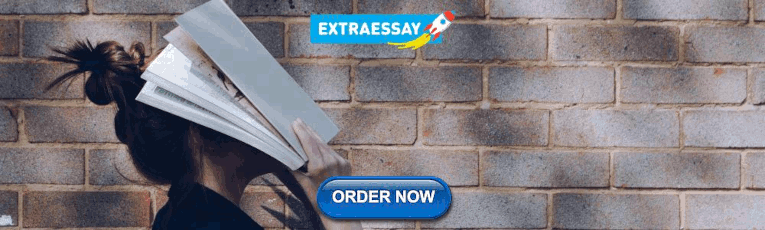
IMAGES
VIDEO
COMMENTS
Historically, natural products (NPs) have played a key role in drug discovery, especially for cancer and infectious diseases 1, 2, but also in other therapeutic areas, including cardiovascular ...
This review is an updated and expanded version of the five prior reviews that were published in this journal in 1997, 2003, 2007, 2012, and 2016. For all approved therapeutic agents, the time frame has been extended to cover the almost 39 years from the first of January 1981 to the 30th of September 2019 for all diseases worldwide and from ∼1946 (earliest so far identified) to the 30th of ...
A literature survey has been carried out to determine the utility of natural molecul … Natural products are used since ancient times in folklore for the treatment of various ailments. Plant-derived products have been recognized for many years as a source of therapeutic agents and structural diversity. ... This review presents a study on the ...
Many natural products have been used as drugs for the treatment of diverse indications. While most U.S. pharmaceutical companies have over the years reduced or eliminated their in-house natural product groups, new approaches for compound screening and chemical synthesis are resurrecting interest in exploring the therapeutic value of natural ...
Natural products (NPs) have been the centre of attention of the scientific community in the last decencies and the interest around them continues to grow incessantly. As a consequence, in the last 20 years, there was a rapid multiplication of various databases and collections as generalistic or thematic resources for NP information. In this review, we establish a complete overview of these ...
Journal scope. Natural Product Reports (NPR) is a critical review journal that stimulates progress in all areas of natural products research, including isolation, structural and stereochemical determination, biosynthesis, biological activity and synthesis.The scope of the journal is very broad, and many reviews discuss the role of natural products in the wider bioinorganic, bioorganic and ...
This review demonstrates the utility of natural products in the development of drugs for human use, and explores how, over the years, natural-product-based drugs have led to very significant advances in the treatment of human diseases. The examples presented range from instances where the actual drug was a pure natural product, through to ...
Natural products (NPs) and their derivatives have been recognized for many years as a source of therapeutic agents. NPs are biologically active substances originating from natural sources such as plant species, animals, and microbes (Baker et al., 2007).Fig. 1 illustrates that they are segmented into four categories depending on respective biosynthetic sources.
Nature is considered a prolific source of diverse biologically active chemotypes. While most reviews have focused on the characteristics of the chemical backbones of natural products (NPs), few have tried to provide an overview of their origins (the living organisms in which they are produced), chemical classes, and biological activities. This review discusses the current knowledge on NP ...
Natural products and their synthesis have always fascinated organic chemists, frequently providing the inspiration and testing ground for new synthetic methods. This Review considers examples of ...
This review reports on recent strategies in developing nanodelivery systems for natural products, according to their classification as polymeric-based and lipid-based nanovectors (Fig. 2), evidencing their characteristics, advantages and limitations.Specifically, polymeric-based nanocarriers include polymeric nanoparticles, among which nanospheres and nanocapsules, polymeric micelles and ...
Natural product is one of the sources of drugs in pharmaceutical industry, and one of the notable origin of natural product is the medicinal plants. ... Electronic literature review method was used in the study. The data were collected from different databases such as Google, Medline, PubMed, Science Direct, ...
Explore the latest full-text research PDFs, articles, conference papers, preprints and more on NATURAL PRODUCT DRUG DISCOVERY. Find methods information, sources, references or conduct a literature ...
Natural products are an important source of new drugs or serve as templates for the development of new synthetic drugs, from anticancer therapies to antibiotics. A significant number of natural product drugs are actually produced by microbes or through their interaction with hosts (Newman and Cragg, 2020). One of the main reasons for exploring ...
This review provides an update on the current taxonomic classification of the Lithistida, describes structures and biological activities of 131 new natural products, and discusses highlights from the total syntheses of 16 compounds from marine sponges of the Order Lithistida providing a compilation of the literature since the last review ...
Covering: January to December 2021. This review covers the literature published in 2021 for marine natural products (MNPs), with 736 citations (724 for the period January to December 2021) referring to compounds isolated from marine microorganisms and phytoplankton, green, brown and red algae, sponges, cnidarians, bryozoans, molluscs, tunicates, echinoderms, mangroves and other intertidal ...
About Natural Product Reports. High impact, critical reviews in natural products research and related areas Synopses for proposed articles are considered by the Editorial Board Editorial Board Chair: Tobias Gulder Impact Factor: 11.9 Time to first decision (peer reviewed): 42.5 days
About the journal. Royal Society of Chemistry. This journal is part of a pilot that enables researchers to search and browse over 70,000 articles in 35 journals from four other participating publishers alongside Elsevier-published content on ScienceDirect.
This study aims to review the literature on the relationship among natural products, traditional medicines, and modern medicine, and to explore the possible concepts and methodologies from natural products and traditional medicines to further develop drug discovery. The unique characteristics of theory, application, current role or status, and ...
Natural products represent major approach for the discovery and development of new drugs. An updated review on the natural products is lacking in the literature. So, in the present review, an attempt has made to pool the source of current natural product information for health care professionals, to update the detailed
The synthesis of natural products plays such an important role in organic and biological chemistry that it is surprising that none of the previous 87 thematic issues of Chemical Reviews have been devoted to the subject. Since this is one of the oldest and most tradition-rich fields of chemistry, and the topic of innumerable monographs and compendia, it is difficult to offer any new or not oft ...
This review covers the literature published in 2019 for marine natural products (MNPs), with 719 citations (701 for the period January to December 2019) referring to compounds isolated from marine microorganisms and phytoplankton, green, brown and red algae, sponges, cnidarians, bryozoans, molluscs, tunicates, echinoderms, mangroves and other intertidal plants and microorganisms.
Abstract. Covering: January to the end of December 2022. This review covers the literature published in 2022 for marine natural products (MNPs), with 645 citations (633 for the period January to December 2022) referring to compounds isolated from marine microorganisms and phytoplankton, green, brown and red algae, sponges, cnidarians, bryozoans ...