Tomato (Solanum lycopersicum): A Model Fruit-Bearing Crop
Affiliation.
- 1 Department of Plant Biology, University of California, Davis, CA 95616, USA.
- PMID: 21356708
- DOI: 10.1101/pdb.emo105
INTRODUCTIONTomato (Solanum lycopersicum) is one of the most important vegetable plants in the world. It originated in western South America, and domestication is thought to have occurred in Central America. Because of its importance as food, tomato has been bred to improve productivity, fruit quality, and resistance to biotic and abiotic stresses. Tomato has been widely used not only as food, but also as research material. The tomato plant has many interesting features such as fleshy fruit, a sympodial shoot, and compound leaves, which other model plants (e.g., rice and Arabidopsis) do not have. Most of these traits are agronomically important and cannot be studied using other model plant systems. There are 13 recognized wild tomato species that display a great variety of phenotypes and can be crossed with the cultivated tomato. These wild tomatoes are important for breeding, as sources of desirable traits, and for evolutionary studies. Current progress on the tomato genome sequencing project has generated useful information to help in the study of tomato. In addition, the tomato belongs to the extremely large family Solanaceae and is closely related to many commercially important plants such as potato, eggplant, peppers, tobacco, and petunias. Knowledge obtained from studies conducted on tomato can be easily applied to these plants, which makes tomato important research material. Because of these facts, tomato serves as a model organism for the family Solanaceae and, specifically, for fleshy-fruited plants.
- Open access
- Published: 20 September 2022
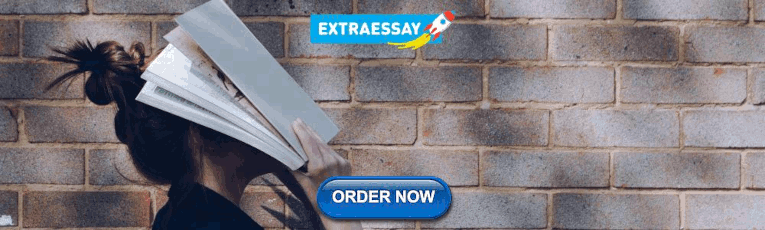
Tomato molecular biology – special collection of papers for molecular horticulture
- Graham B. Seymour 1 &
- Jocelyn K. C. Rose 2
Molecular Horticulture volume 2 , Article number: 21 ( 2022 ) Cite this article
2847 Accesses
1 Citations
2 Altmetric
Metrics details
Tomato ( Solanum lycopersicum ) is the second most important vegetable crop globally, after potato, with about 100 million tons fresh fruit being grown on 3.7 million hectares (FAO 2021 ), and is of great importance in the human diet due to the large amount of fruit consumed. The fruit are eaten both fresh and, equally importantly, as a processed product in puree, soups and canned products. They provide an important dietary source of vitamins and minerals, such as K, Fe and Ca, and are known for the large number of health promoting secondary metabolites, including the carotenoid and flavonoid pigments that give the fruit a spectrum of yellow, red and orange colours.
Tomato commercial production and breeding is supported by a long history of research that has led to the identification of regions of the tomato genome that control a host of important traits including disease resistance, yield and fruit quality. The first tomato genome was sequenced a decade ago and the sequence is now in its fifth iteration with 100 s of genome sequences from a wide spectrum of wild species crop relatives and cultivated varieties. The diploid nature of tomato, genomic resources and a wide range of single gene mutants make it an excellent model plant to study dicot crop species and especially those with fleshy fruits.
In this special collection, we bring together a range of papers to explore the latest developments and scientific insights in tomato molecular biology. This brief editorial summarises the key features of the papers that collectively set the scientific discovery in the context of plant development and highlight relevance to horticulture.
Plant architecture is a critical determinant of crop productivity and influences, among other factors, the number of reproductive shoots. The hormonal control of plant architecture has been the subject of studies for well over 100 years, but recent advances in genomics have accelerated our understanding of its molecular basis. Tomato is an especially useful model to investigate the association between plant architectural features and their impact on crop performance. Side branching in tomato is undesirable and results in unwanted labour and management costs. Three main phytohormone classes are known to influence side branching: auxins, cytokinins (CKs) and strigolactones. Auxin inhibits the outgrowth of axillary buds and maintains apical dominance. CKs act antagonistically to auxin, suppressing apical dominance and allowing release of axillary buds from dormancy. Auxin modulates CK concentration by repressing its levels, while strigolactones affect bud inhibition by modulating auxin transport. In addition, there seems to be some interplay between CK and strigolactone levels, and the overall picture highly complex. In this special issue, Pino et al. ( 2022 ) report that tomato plants overexpressing the cytokinin-deactivating gene CYTOKININ OXIDASE 2 ( CKX2 ) showed excessive growth of axillary shoots, which is opposite to the phenotype expected of plants with reduced CK content. The authors suggest that CKs cause their paradoxical effects on branching by disturbing auxin status, and by altering the expression of genes associated with branching and CK homeostasis. The study highlights the intricacy of the molecular control of side branching, and the importance of this research in understanding the control of plant architecture for crop improvement.
The architecture of plant roots is as important as that of above ground organs and this is an area of particular interest to growers of grafted vegetable crops. Many Solanaceous crops, including tomato, pepper and eggplant, are grown as elite scion genotypes grafted onto to superior performing rootstocks, and this is especially widespread for commercial tomato production in Europe and the USA. The root stocks can be chosen to enhance scion resistance to diseases and abiotic stress, involving conditions such as low nutrient availability and high salinity. The genetic basis of root traits in tomato and other crops is relatively poorly understood and this knowledge is an important prerequisite for a rational approach to breeding for improved root traits. In this issue, Kevei et al. ( 2022 ) report their work on a tomato mutant, bushy root-2 ( brt-2 ), which has a twisting tap root and a high density of lateral roots, giving a bushy appearance. These lateral roots are also abnormal in that they twist and curl, and plant growth is slower than that of wild type. The brt-2 candidate gene was identified by genetic mapping as a class B heat shock factor protein encoded by SolycHsfB4a. Whole genome resequencing and SNP (single nucleotide polymorphism) and KASP (Kompetitive allele specific PCR) markers were used to fine map the brt-2 gene and a SNP was identified as a strong candidate for the causal brt- 2 mutation. The authors discuss reasons why such a mutation could influence the function of the protein encoded by the gene at the brt-2 locus and how this might result in the bushy root phenotype. The study provides an important extension to our knowledge of root architecture in tomato. Moreover, since a related Arabidopsis thaliana gene, ATHSFB4 , is induced by root knot nematode (RKN) infection, and its loss-of-function mutants are resistant to RKNs, BRT-2 could be a target gene for RKN resistance, an important trait in tomato rootstock breeding.
Studies have shown that the action of auxin, CKs, and gibberellins (GAs) can reduce plant resistance to water deficiency, and there is evidence that inhibition of GA activity can enhance plant performance under stress conditions. In this collection, Shohat et al. ( 2021 ) review the importance of GAs in tomato, including in regulating responses to abiotic stresses, such as drought. They authors consider how drought affects GA biosynthesis and signalling in tomato and discuss possible ways in which knowledge of GA pathways could be used to generate drought tolerant plants. These include interrupting GA binding to its receptor, GID1, altering the effectiveness of the downstream signalling pathway and, perhaps most promisingly, through deactivation of GA itself.
Irregular watering and other factors can result in devastating losses in commercial tomato operations due to a physiological disorder known as blossom-end rot (BER). Typical symptoms of BER appear as small, light-coloured, water-soaked spots on the blossom end of the fruit, which is associated with cell plasmolysis and leaky membranes. After BER induction, BER-affected areas often expand in the form of brown necrotic regions covering a significant proportion of the fruit and, in some extreme cases, can affect the entire fruit. The condition affects tomato, but also many other fruits, including pepper ( Capsicum annuum L.), watermelon ( Citrullus lanatus (Thunb.) and eggplant ( Solanum melongena L.). In this special collection, Topcu et al. ( 2022 ) review the latest information of the biological basis of BER as well as the genetic and molecular underpinnings of this important physiological disorder. The paper presents information on the role of altered Ca 2+ homeostasis among different cellular compartments, and especially the role of Ca 2+ and interactions with pectin in the fruit primary cell walls. Other important factors include reactive oxygen species (ROS), which appear to be a critical component of BER development and are linked to changes in Ca 2+ deficiency. Tomato varieties show variation in their susceptibility to BER, which suggests a possible underlying genetic basis for the condition. Genetic mapping and differential expression analysis has uncovered possible candidate BER-associated genes, but further work is needed to develop genetic approaches to prevent this physiological disorder.
Tomato fruit are the most widely used model to investigate the developmental regulation of ripening in fleshy fruited species, and there is a deep set of resources to help dissect the biochemical, molecular, and genetic events linked to tomato fruit ripening control. In this special collection, Zhu et al. ( 2021 ) review the metabolic changes that effect fruit quality during tomato ripening. The review focuses on the transcriptional and post-translational control of the networks that affect the accumulation of important biochemical components in fruit tissues, from pigments and sugars through to other metabolites with health promoting properties. The review links the biochemistry of the ripening process with the key genes underlying these processes and the associated quality traits.
One of the key processes determining fruit quality is the rate of softening and tomato again represents one of the model organisms where this process has been researched in some considerable depth. The review in this collection by Wang and Seymour ( 2022 ) summarises the most recent data available on the control of softening in tomato. The authors first describe the hormonal cues, epigenetic priming and transcriptional control linked with the softening process. These areas of research are still fragmented and there is a limited understanding of the interrelationship between such high-level events. However, the actual biochemical changes involved in texture changes are a little clearer, although the specific timing and role of particular biochemical events still generally remain obscure. In essence, it seems that modification of pectic polysaccharides plays a major role in softening through the action of enzymes such as pectate lyase (PL), polygalacturonase (PG) and several other activities. Gene editing of tomato to silence these genes leads to an inhibition of softening in the case of PL, although it is not the only factor involved in textural changes and studies of the transcriptional control of softening are providing new insights. Understanding the molecular basis of softening is providing targets for molecular breeding that will likely improve shelf life, and possibly fruit that are more resilient to fungal and bacterial spoilage.
A primary barrier to pathogen invasion and spoilage is the fruit cuticle. In this issue Bres et al. ( 2022 ) describe how they screened a mutant collection of the miniature tomato cultivar Micro-Tom for fruit cracking mutants and found a mutant with a glossy fruit phenotype. The authors then used a mapping-by-sequencing strategy to identify the causal mutation as an amino acid change in the SlSHN2 transcription factor, which is specifically expressed in outer epidermis of growing fruit. The mutation has a marked effect on cuticle composition. In addition to the direct effects on cuticle formation and composition, the mutation resulted in a wide range of other gene expression changes that link the SlSHN2 gene to coordination of cuticle deposition, epidermal patterning and defence against biotic and abiotic stresses.
New insights into the molecular control of plant growth and fruit ripening will likely come with a better understanding of the role of genome structural variation and epigenetics in controlling gene expression. The paper in this special collection by Jobson and Roberts ( 2022 ) reviews the current understanding of genomic structural variation (SV) in tomato and its role in plant immunity. Structural variation for the purpose of this review is defined as changes that range from greater than 30 base pairs to several megabases. These can include inversions, duplications and deletions. The authors discuss the various molecular mechanisms leading to common structural changes. Drawing on information from several species, including tomato, they then discuss identification of SVs in plant genomes. The main part of the review focuses on the potential roles of SV in responses to biotic and abiotic stress. The authors conclude with a section on engineering immunity in tomato using SVs.
Other articles published in Molecular Horticulture, outside this current collection that may also be of interest to readers include those on tomato fruit size control by a zinc finger protein regulating pericarp cell size (Zhao et al. 2021 ), tomato SlRUP as a negative regulator of UV-B photomorphogenesis (Zhang et al. 2021 ) and genome-wide binding analysis of the tomato transcription factor SlDof1 and its regulatory impacts on fruit ripening (Wang et al. 2021 ).
The papers in this special collection highlight a broad range of exciting discoveries, technology platforms and resources that illustrate the tremendous and growing significance of tomato as an experimental model, and socially important crop. The future for tomato research remains bright!
Bres C, Petit J, Reynoud N, et al. The SlSHN2 transcription factor contributes to cuticle formation and epidermal patterning in tomato fruit. Mol Hortic. 2022;2:14. https://doi.org/10.1186/s43897-022-00035-y .
Article CAS Google Scholar
FAO World Food and Agriculture - Statistical Yearbook 2021 ( https://www.fao.org/ ).
Google Scholar
Jobson E, Roberts R. Genomic structural variation in tomato and its role in plant immunity. Mol Hortic. 2022;2:7. https://doi.org/10.1186/s43897-022-00029-w .
Kevei Z, Ferreira SDS, Casenave CMP, et al. Missense mutation of a class B heat shock factor is responsible for the tomato bushy root-2 phenotype. Mol Hortic. 2022;2:4. https://doi.org/10.1186/s43897-022-00025-0 .
Pino LE, Lima JE, Vicente MH, et al. Increased branching independent of strigolactone in cytokinin oxidase 2-overexpressing tomato is mediated by reduced auxin transport. Mol Hortic. 2022;2:12. https://doi.org/10.1186/s43897-022-00032-1 .
Shohat H, Eliaz NI, Weiss D. Gibberellin in tomato: metabolism, signaling and role in drought responses. Mol Hortic. 2021;1:15. https://doi.org/10.1186/s43897-021-00019-4 .
Topcu Y, Nambeesan SU, van der Knaap E. Blossom-end rot: a century-old problem in tomato (Solanum lycopersicum L.) and other vegetables. Mol Hortic. 2022;2:1. https://doi.org/10.1186/s43897-021-00022-9 .
Wang D, Seymour GB. Molecular and biochemical basis of softening in tomato. Mol Hortic. 2022;2:5. https://doi.org/10.1186/s43897-022-00026-z .
Wang Y, Wang P, Wang W, et al. Genome-wide binding analysis of the tomato transcription factor SlDof1 reveals its regulatory impacts on fruit ripening. Mol Hortic. 2021;1:9. https://doi.org/10.1186/s43897-021-00011-y .
Zhang C, Zhang Q, Guo H, et al. Tomato SlRUP is a negative regulator of UV-B photomorphogenesis. Mol Hortic. 2021;1:8. https://doi.org/10.1186/s43897-021-00010-z .
Zhao F, Zhang J, Weng L, et al. Fruit size control by a zinc finger protein regulating pericarp cell size in tomato. Mol Hortic. 2021;1:6. https://doi.org/10.1186/s43897-021-00009-6 .
Zhu F, Wen W, Cheng Y, et al. The metabolic changes that effect fruit quality during tomato fruit ripening. Mol Hortic. 2021;2:2. https://doi.org/10.1186/s43897-022-00024-1 .
Download references
Author information
Authors and affiliations.
School of Biosciences, Division of Plant and Crop Science, University of Nottingham, Loughborough, Leics, LE12 5RD, UK
Graham B. Seymour
School of Integrative Plant Science, Cornell University, 331 Emerson Hall, Ithaca, NY, 14853, USA
Jocelyn K. C. Rose
You can also search for this author in PubMed Google Scholar
Contributions
The authors read and approved the final manuscript.
Authors’ information
Graham Seymour and Jocelyn Rose are Associate Editors of Molecular Horticulture and the editors for the ‘Tomato Molecular Biology’ special collection.
Corresponding author
Correspondence to Graham B. Seymour .
Ethics declarations
Competing interests.
The authors declare that they have no competing interests.
Additional information
Publisher’s note.
Springer Nature remains neutral with regard to jurisdictional claims in published maps and institutional affiliations.
Rights and permissions
Open Access This article is licensed under a Creative Commons Attribution 4.0 International License, which permits use, sharing, adaptation, distribution and reproduction in any medium or format, as long as you give appropriate credit to the original author(s) and the source, provide a link to the Creative Commons licence, and indicate if changes were made. The images or other third party material in this article are included in the article's Creative Commons licence, unless indicated otherwise in a credit line to the material. If material is not included in the article's Creative Commons licence and your intended use is not permitted by statutory regulation or exceeds the permitted use, you will need to obtain permission directly from the copyright holder. To view a copy of this licence, visit http://creativecommons.org/licenses/by/4.0/ . The Creative Commons Public Domain Dedication waiver ( http://creativecommons.org/publicdomain/zero/1.0/ ) applies to the data made available in this article, unless otherwise stated in a credit line to the data.
Reprints and permissions
About this article
Cite this article.
Seymour, G.B., Rose, J.K.C. Tomato molecular biology – special collection of papers for molecular horticulture. Mol Horticulture 2 , 21 (2022). https://doi.org/10.1186/s43897-022-00042-z
Download citation
Published : 20 September 2022
DOI : https://doi.org/10.1186/s43897-022-00042-z
Share this article
Anyone you share the following link with will be able to read this content:
Sorry, a shareable link is not currently available for this article.
Provided by the Springer Nature SharedIt content-sharing initiative
Molecular Horticulture
ISSN: 2730-9401
- Submission enquiries: [email protected]
Click through the PLOS taxonomy to find articles in your field.
For more information about PLOS Subject Areas, click here .
Loading metrics
Open Access
Peer-reviewed
Research Article
Tomato plants ( Solanum lycopersicum L.) grown in experimental contaminated soil: Bioconcentration of potentially toxic elements and free radical scavenging evaluation
Roles Conceptualization, Data curation, Writing – original draft
Affiliation Dipartimento di Scienze e Tecnologie Ambientali, Biologiche e Farmaceutiche, Università della Campania “Luigi Vanvitelli”, Caserta, Italy
Roles Conceptualization, Investigation, Supervision, Writing – review & editing
* E-mail: [email protected]

Roles Conceptualization
Affiliation Consiglio Nazionale delle Ricerche, Istituto di Chimica Biomolecolare, Pozzuoli, Italy
Roles Conceptualization, Formal analysis, Methodology, Supervision, Writing – review & editing
Roles Data curation, Methodology, Writing – original draft
- Concetta Piscitelli,
- Margherita Lavorgna,
- Rocco De Prisco,
- Elio Coppola,
- Eleonora Grilli,
- Chiara Russo,
- Marina Isidori
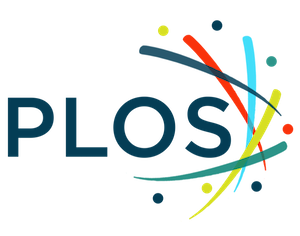
- Published: August 13, 2020
- https://doi.org/10.1371/journal.pone.0237031
- Reader Comments
Tomato is the most widespread vegetable crop in the world. In Italy, tomatoes are mainly cultivated in the South and in the Campania region, precisely in the area called Agro Nocerino-Sarnese. This flatland is affected by an extreme level of environmental degradation, especially related to the Sarno River, where concentrations of Potential Toxic Elements (PTEs) have been found to be higher than the maximum permitted level. The aim of this study was to determine the PTEs uptake by roots and their translocation to the aerial parts of the plants of two cultivars of tomatoes (Pomodoro Giallo and San Marzano Cirio 3). To the purpose, samples of the two cultivars were grown both in pots with experimentally contaminated soil containing: Cr or Cd or Pb at extremely high concentrations and in pots with uncontaminated soils (control). Additionally, the antioxidant properties of the cultivars selected grown on uncontaminated/contaminated soils were assessed. The results showed that Cd was the contaminant that most significantly interfered with the growth of both cultivars of tomato plants, whereas Pb caused lower phenotypical damage. Cd translocation from root to the organs of tomato plants was observed in both cultivars. Specifically, the total amount of Cd found in stems and leaves was higher in the Pomodoro Giallo (254.4 mg/kg dry weight) than in the San Marzano Cirio 3 (165.8 mg/kg dry weight). Cd was the only PTE found in the fruits of both cultivars, with values of 6.1 and 3.9 mg/kg dry weight of Pomodoro Giallo and San Marzano Cirio 3, respectively. The fruits of tomato plants grown in PTEs-contaminated soil showed inhibition or stimulations of the radical scavenging activity compared to the fruits grown in uncontaminated soil. This study highlighted that, despite the relatively high experimental concentrations of PTEs, their translocation to the edible part was comparatively low or absent.
Citation: Piscitelli C, Lavorgna M, De Prisco R, Coppola E, Grilli E, Russo C, et al. (2020) Tomato plants ( Solanum lycopersicum L.) grown in experimental contaminated soil: Bioconcentration of potentially toxic elements and free radical scavenging evaluation. PLoS ONE 15(8): e0237031. https://doi.org/10.1371/journal.pone.0237031
Editor: Branislav T. Šiler, Institute for Biological Research "S. Stanković", University of Belgrade, SERBIA
Received: March 30, 2020; Accepted: July 17, 2020; Published: August 13, 2020
Copyright: © 2020 Piscitelli et al. This is an open access article distributed under the terms of the Creative Commons Attribution License , which permits unrestricted use, distribution, and reproduction in any medium, provided the original author and source are credited.
Data Availability: All relevant data are within the manuscript.
Funding: This work was supported by the University of Campania “Luigi Vanvitelli” through the VALERE program. The funders had no role in study design, data collection and analysis, decision to publish, or preparation of the manuscript.
Competing interests: The authors have declared that no competing interests exist.
Introduction
The cultivated tomato, Solanum lycopersicum L. endemic to Central and South America, belongs to the family Solanaceae. It is the world’s most highly grown vegetable due to its status as a basic ingredient in a large variety of raw, cooked or processed foods.
Tomato is cultivated in tropical and temperate climates, especially in Mediterranean countries such as Spain and Italy [ 1 ]. Nowadays, tomato consumption has increased due to its richness in bioactive compounds such as antioxidant molecules (i.e. carotenoids, flavonoids, vitamins and tocopherols) known for their beneficial effects on human health [ 2 ].
However, environmental factors as temperature, and light as well as fertilization of the soil play a key role in the richness of bioactive compounds in different cultivars as well as fruit ripeness [ 3 – 5 ]. Piscitelli et al. [ 6 ] focused on San Marzano Cirio 3 and Pomodoro Giallo cultivars while studying the antioxidant and antitumoral properties of nine different cultivars of tomatoes. The authors selected those cultivars for their radical scavenging activity (high polyphenolic content) and their antitumoral properties (content of lycopene and carotene). In Italy, tomatoes are mainly cultivated in the South especially in Agro Nocerino Sarnese (Campania Region) since it is one of the most fertile Italian flatlands for tomato production (31x10 6 kg of tomatoes in open fields and 44x10 6 kg tomatoes production and processing in open fields) [ 7 ].
This area is unfortunately affected by adverse environmental factors in relation to the Sarno River and its tributaries. The Sarno River is indeed, the most polluted river in Europe due to anthropogenic activity and uncontrolled industrial and urban waste disposal [ 8 , 9 ]. Many studies have shown that the Sarno river is contaminated by Potentially Toxic Elements (PTEs). Montuori and co-authors [ 10 ] demonstrated that this river contributes to the pollution of the Tyrrhenian Sea with metals and polycyclic aromatic hydrocarbons (PHA). In a study conducted by Parrella et al. [ 9 ], it is stated that the porewaters of the Sarno basin showed mutagenic/genotoxic effects. Recently, Cicchella and collaborators [ 11 , 12 ] demonstrated that Sarno stream sediments are contaminated with chromium (Cr) from tanneries and from copper (Cu) released from agricultural activities.
The habit of irrigating crops using river waters has been restricted from the nineties because of the heavily polluted wastewater produced in the tanning industry improperly dumped into the river and its tributaries along a short riverbed (24 Km). Nevertheless, the soil may be contaminated by frequent flooding of the Sarno river, and soil pollution has become a major concern since pollutants persist in this compartment longer than in any other environmental compartment [ 13 ]. These pollutants may be absorbed by roots and accumulated at high concentrations in the edible parts of vegetables, thus reaching animals and humans through the trophic chain causing remarkable concern for human health [ 14 – 16 ].
Two studies have focused on the transfer of PTEs from contaminated soil to vegetables grown in the Sarno area [ 14 , 15 ], indicating the exposure to multiple trace elements with additive, antagonistic, and/or synergistic effects (differently contributing to the potential PTEs translocation).
In contrast with findings from previous studies, the aim of this study was to detect the concentrations of Cr, Cd or Pb occurring in two cultivars grown in pots with experimentally contaminated soil. The PTEs (Cr, Cd or Pb) were selected based on the most occurring heavy metals in the Sarno basin area specifically Cr and Pb occurring in Agro Nocerino Sarnese soil at concentrations higher than the maximum permitted concentrations (152/2006 limits: 150 and 100 mg/kg dry weight, respectively for Cr and Pb) [ 17 ]. Cd was considered adequate for its ability of translocation into the fruits of the plants [ 14 ]. Thus we assessed the uptake by roots and their translocation to the aerial parts of the plants especially the edible parts. Additionally, the potential modifications in the antioxidant properties of the cultivars selected (Pomodoro Giallo and San Marzano Cirio 3) were assessed. These cultivars were selected on the basis of their high radical scavenging activity, already found in our previous study [ 6 ].
Materials and methods
The experimental plan was conducted in phases proceeding initially with analysis of the physico-chemical properties of the soil to plant the seedlings of two tomato cultivars. Then, the soil was experimentally contaminated in pots with aqueous mono-metal solution of Cd, Cr, Pb (to exclude any environmental pollution, adequate disposal procedures for hazardous residues were followed). On fruit maturation, the roots, stems, leaves and fruits were collected, lyophilized and mineralized to determine the PTE concentrations in the single parts. Only the edible part of the two cultivars was subjected to extraction for free radical activity evaluation.
Soil analyses
The soil used for PTE contamination was collected in Mondragone 41° 05' 43.2" N—13° 54' 38.5" E, along the Domitio coastline (in Campania Region) at 0–20, 20–40 cm depth (Eutric Arenosols, WRB-SR, 2015). The sample underwent physico-chemical analyses performed on air-dried 2 mm soil (Fine-Earth fraction, f.e.) in accordance with procedures authorized by the Ministero delle Politiche Agricole e Forestali [ 18 ]. Soil texture was determined using the pipette method, while soil pH was measured potentiometrically on 1:2.5 soil to water (w/v). Soil electrical conductivity (EC) was measured on 1:5 soil to water (w/v). Organic carbon (OC) was determined according to the Walkley–Black oxidation method and cation exchange capacity (CEC) was measured by barium chloride and triethanolamine (BaCl 2 ‐TEA) method at pH 8.2.
Pot experiments
Seeds of Solanum lycopersicum L. cultivars Pomodoro Giallo and San Marzano Cirio 3, were soaked for 15 minutes in tap water and sterilized with sodium hypochlorite (5%) for 15 min. Then, they were washed three times with sterile water and sown in glass beakers with sterile soil. Seeds were exposed to sunlight to germinate at the end of March in a greenhouse. After 30 days the seedlings were transferred into open air to grow in different experimental pots containing 2 kg of the selected soil irrigated with 125 mL of mono-metal solutions for 12 days at increasing percentages equal to 5, 10 and 20% of Cd, Cr and Pb, based on the soil CEC value obtained (cmol(+)/kg f.e.). For each cultivar and for each aqueous solution of PTE at different percentages, three replicates were set up. During cultivation, water was systematically added to soil in pots (maximum 125 mL).
The aim was to simulate growth in an extremely contaminated environment as suggested by Buondonno et al. [ 19 ]. The contamination was performed following transplantation to guarantee plant rooting and greater growth potential. For the contamination of the soil, an aqueous solution of cadmium chloride (CdCl 2 ) was used as source of Cd; an aqueous solution of chromium hydroxide sulfate [Cr 4 (SO 4 ) 5 (OH) 2 ] as source of Cr and an aqueous solution of lead nitrate [Pb(NO 3 ) 2 ] as source of Pb. The different content of SO 4 2- and NO 3 —were equilibrated with addition of KNO 3 and K 2 SO 4 to the soil. For each percentage of PTEs (5, 10 and 20%), a respective negative control (PG-NC and PR-NC) was used namely tomato plants (PG and PR) grown in uncontaminated soil, irrigated with water and supplemented with KNO 3 and K 2 SO 4 at the percentages of 5, 10 and 20%. The amounts of the compounds added to the soil are reported in Table 1 .
- PPT PowerPoint slide
- PNG larger image
- TIFF original image
https://doi.org/10.1371/journal.pone.0237031.t001
To ensure the radical part as the only experimental source of PTEs, and to exclude the uptake of PTEs from polluted air by absorption through above- ground parts, the tomato plants of the two selected cultivars were grown in an area characterized by the absence of emissions from mobile sources related to vehicle transport and fuels (linear emission), and in absence of emission related to the processes of energy combustion of fuels, industrial processes, discharging substances into the air through point emissions. On fruit maturation, the single parts of the tomato plant (roots, stems, leaves and fruits) were collected. Fruits were cleaned using deionized water to remove dust and soil, while roots were gently shacked to remove the soil attached. The samples derived from the three replicates of each part of the cultivar plants were stored in polyethylene bags for transport to the lab at a constant temperature in dry ice, and stored at -80°C.
Chemical analysis for PTEs concentrations in tomato fruits, leaves, stems and roots
To detect the concentration of the PTEs [ 20 , 21 ], in fruits, leaves, stems and roots of PG and PR plants, the samples were lyophilized (- 150 mTorr, - 80°C, 96 h) and then mineralized with hydrogen peroxide (H 2 O 2 ) and nitric acid (HNO 3 ). The mineralization method consisted in a digestion with hot concentrated acid in closed containers in a diffuse microwave oven (Microwave Digestion System, Start D Model, Milestone) to dissolve metals associated with particles present in colloidal and/or organic forms. Using the mineralization program ( Table 2 ), 2 mL of H 2 O 2 and 6 mL of HNO 3 were added to 250 mg of pulverized tomato fruits, leaves, stems and root plant material. The tubes were placed in the microwave for 22 min.
https://doi.org/10.1371/journal.pone.0237031.t002
After the mineralization cycle, the samples were diluted with 50 mL of distilled water, filtered and analyzed by plasma emission spectrometry (ICP-OES) by a ICP-MS (AGILENT, 7500ce).
The concentrations of the selected PTEs found in the negative controls were below the instrumental detection threshold of the ICP-OES analysis (Cr < 0.1 ppm, Pb from 0.1 to 1 ppm, Cd from 0.1 to 1 ppm). The quality control for accuracy and precision of the method was performed with standard reagents for ICP-MS.
Moreover, to assess the environmental impact linked to soil contamination with PTEs, the following parameters were considered-the Bioconcentration Factor (BCF), which is the ratio between the concentration of a PTE detected in plant roots and the concentration of the same PTE detected in the soil; this parameter is an index of the potential absorption of the PTE from the soil to the plant. A BCF value greater than 1 indicates that the element is concentrated in the plant roots [ 22 ]; and the Translocation Factor (TF), equal to the ratio between the concentration of a PTE detected in the above-ground parts of the plant and the concentration of the same PTE detected in the roots; this factor refers to the ability of the plant to transfer PTE from roots to stems, leaves, fruits and seeds and may provide information both on the risk of contamination of the edible parts of the plant and on its potential use in phytoremediation techniques.
Data analysis
The results from three independent experiments were expressed as mean values ± standard error. Statistical analysis was performed by Graphpad Prism5 using 2way-ANOVA , Bonferroni . Differences were considered significant when*p<0.05, **p<0.01. ***p<0.001.
Free radical scavenging assays
The free-radical scavenging capacity was evaluated by ABTS and DPPH assays on tomato fruit extracts. Tomato fruits were lyophilized and pulverized before extraction. The extraction was then conducted by a solid/liquid ratio of 2:1:1 in which the solvent was a mixture of chloroform, methanol and water, obtaining two fractions: a hydroalcoholic and a lipophilic fraction. The hydroalcoholic fraction was further separated into hydrophilic and methanolic fractions by means of Sep-pak ® C18 cartridges. Subsequently, the extracts were filtered and concentrated in a rotary evaporator in vacuum and dried under N 2 . For analysis, the hydrophilic, methanolic and chloroformic extracts obtained were dissolved in water, methanol and chloroform, respectively. The methanolic and chloroform extract stock solutions did not exceed the 5% of methanol and 2% of chloroform.
The protocol described by Re et al. and Lavorgna et al. [ 23 , 24 ] with some modifications was followed. The ABTS radical was generated by adding the potassium persulphate (140 mM) to the ABTS solution (7 mM) for 12–16 h in the dark. Then, the solution was diluted reaching the absorbance of 0.7 ± 0.2 OD at 734 nm. When an antioxidant substance is added to the ABTS solution, the radical is reduced with a consequent discoloration of the reaction mixture.
The outcomes from three independent experiments were expressed as EC50 (the median effective concentration of sample able to reduce the initial absorbance of the ABTS and DPPH radical) values. The EC50 values were obtained by Graphpad Prism5 analysis by non-linear regression (log agonist vs. normalized response-variable slope) with 95% confidence range. The results were analyzed by the unpaired t-test . Differences were considered as significant when p values were *p<0.05 and **p<0.01.
The soil showed a sandy texture and the results of physicochemical analyses revealed a moderately alkaline pH (8.0, in H2O). The electrical conductivity was 0.48 dS/m, the OC was 17.2 g/kg while CEC value was 17.1 (cmol(+)/kg).
At harvest time, Pomodoro Giallo and San Marzano Cirio 3 plants, grown in experimental contaminated soils, showed clear phenotypical alterations ( S1 and S2 Figs). These changes were especially evident for the biomass, expressed as dry weight of the plants. The dry weight of PG plants with 20% of Cd, Cr and Pb (based on the soil CEC value obtained, cmol (+) /kg f.e.) was 85%, 53% and 32%, respectively, less than the dry weight of the negative control (136.5g). The dry weight of PR plants with 20% of Cd, Cr and Pb was 82%, 48% and 23%, respectively, less than the dry weight of the respective negative control (102.7g). Among all PTEs, Cd was the main contaminant able to interfere with the growth of the plants of both tomato cultivars. Specifically, at Cd 20%, Pomodoro Giallo plants did not produce fruits, while the San Marzano Cirio 3 plants produced few fruits. As regards Cr, most of the plants showed poor vegetation and scarce fruit production. Finally, among the PTEs tested, Pb was the contaminant responsible for less phenotypical damage to the plants. Moreover, between the two cultivars, Pomodoro Giallo appeared more sensitive to the contamination of the PTEs.
ICP-OES analysis
The concentrations (mg/kg dry weight) at increasing percentages of Cd, Cr and Pb are reported in Table 3 .
https://doi.org/10.1371/journal.pone.0237031.t003
Considering the different dislocation of the PTEs along the plant organs, it is noteworthy that Pb and Cr were found in roots, at the highest tested percentages (20%), in the order of hundreds/thousands and tens of mg/kg dry weight respectively, decreasing to values close to few mg/kg in stems and leaves. Interestingly, Pb was found in roots at 5% at 338.3 and 259 mg/kg in PG and PR, respectively. Furthermore, an increasing PTE%-recovering trend dependent on the quantity of Pb added in the soil was observed, reaching 20%, values equal to 941.8 (PG) and 1572.1 (PR) mg/kg. At the same level (20%) translocation of Cd from root to the organs of tomato plants was observed in both tomato cultivars. Significant differences between PG and PR for each part were observed for *p<0.05, **p<0.01, *** p<0.001. Specifically, the total amount of Cd found in stems and leaves was higher in Pomodoro Giallo (254.4 mg/kg) compared to San Marzano Cirio 3 (165.8 mg/kg).
Table 3 illustrates evident differences in the PTE concentrations of the fruits. Surprisingly, Cd (10%) was the only PTE found in the edible parts of Pomodoro Giallo at 6.1 mg/kg dry weight (about 0.5 mg/kg wet weight) and San Marzano Cirio 3 at 3.9 mg/kg dry weight (about 0.3 mg/ kg wet weight). These concentrations were higher than those recommended by the Commission Regulation (EC) No.629/2008 that sets maximum concentrations for Cd in food stuffs at 0.05 mg/kg wet weight. Concurrently, the Italian legislative decree 152/2006 sets the limit for Cd in the soil at 2 mg/kg dry weight, a significant lower concentration compared to the quantities adopted herein to irrigate the soil, equal to 2 g.
In addition, in the present study, BCF and TF values were calculated in relation to soil Cation-exchange Capacity ( Table 4 ). The PTEs analyzed were not able to concentrate in tomato plant roots (BCF< 1) and only Cd showed the ability to move from roots into the above- ground parts of the tomato cultivars (TF = 4.262 in PG and 1.613 in PR, at 20%). Pomodoro Giallo plants showed a higher translocation potential of Cd from the roots to the above-ground parts of plant than San Marzano Cirio 3 plants, with TF values higher than 1 already at the lowest percentage of Cd used for soil contamination.
https://doi.org/10.1371/journal.pone.0237031.t004
Free radical scavenging evaluation
Measurements of tomato free radical scavenging activity were carried out on the fruits of the tomato plants irrigated at PTE contamination levels of 10% of CEC, the highest level of PTEs resulting in plant fructification.
The results, expressed as EC50 values, are reported in Tables 5 and 6 for Pomodoro Giallo and San Marzano Cirio 3, respectively. It was observed that both tomato fruit extracts coming from uncontaminated and contaminated soils showed antioxidant activity. Among the extracts, the hydrophilic and methanolic extracts showed a higher free-radical scavenging capacity in both ABTS and DPPH assays, compared to chloroformic extracts which presented EC50 values generally higher than the other fractions. In particular, for the DPPH assay, the hydrophilic extracts of PG tomatoes grown on soil contaminated with Cr, showed a significant EC50 value (52.9 mg/L, p<0.01) compared to the negative control EC50 (339.4 mg/L), demonstrating the highest antiradical activity followed by the methanolic extracts (EC50 = 99.7 mg/L, p<0.01) and by the chloroformic extracts (EC50 = 304.1 mg/L, p<0.05) of plants grown in presence of Cd. Conversely, significant increases of EC50 values were observed in both tests, highlighting a reduction of the antiradical scavenging capacity for some fractions of the cultivars exposed to the selected PTEs, especially for PR in the methanolic extracts.
https://doi.org/10.1371/journal.pone.0237031.t005
https://doi.org/10.1371/journal.pone.0237031.t006
Results differed according to the PTEs under analysis; each one showing peculiarities noteworthy of further discussion.
Cd was able to interfere with the growth of both examined tomato cultivar plants. In particular, at 20%, both Pomodoro Giallo and San Marzano Cirio 3 plants showed scarce development, Pomodoro Giallo plants did not produce fruit, while the San Marzano Cirio 3 plants had a low production. These results may be due to the high potential of Cd to be absorbed by roots and accumulated in all the plant organs and tissues, especially in metabolically less active parts of leaves [ 27 ]. The lack or the reduced quantity of fruit observed in the plants exposed to the highest concentration of Cd and Cr was most likely caused by the effect of PTEs on enzymes or other metabolites or by their ability to generate reactive oxygen species which may yield oxidative stress [ 28 ]. In addition, Cr is able to easily modify its oxidation state causing the formation of free reactive oxygen species (ROS) [ 29 , 30 ] which may result in: lipid peroxidation, damage of the chloroplast ultrastructure, reduction of photosynthetic activity or of chlorophyll biosynthesis, reduction of growth and alteration of water balance [ 31 ]. Conversely, some studies have reported that Cr, in the form of Cr (III), is able to increase plant growth at low concentrations [ 32 , 33 ]. Furthermore, this element may produce positive effects on plant growth influencing the production of cytokinins or other growth hormones [ 34 ]. As Cr may have a different impact on plant growth, similarly, plants treated with Cd may or may not show signs of toxicity [ 27 ]. To improve and/or stimulate the growth and development of plants in soils contaminated by Cd, Rao and co-authors [ 35 ] recently suggested ultrasonic seed treatment which increases cadmium tolerance. Finally, among the PTEs tested, Pb was the contaminant that caused less phenotypical damage to the plants. Hu et al. [ 36 ] reported that Pb showed low absorption potential through roots. Differently, Ashraf and Tang [ 37 ] showed that Pb toxicity severely reduced yield and quality- related attributes and plant biomass in two aromatic rice cultivars. In addition to phenotypical responses under stressful environment with PTEs, quantitative analyses were performed on the mineralized samples of the exposed plants. As reported in the results, differences in concentrations of the single PTEs were found in the roots, and in the edible parts of the two cultivars. At the highest percentage of PTE tested, Pb was found mainly in the roots, in the order of hundred/thousands of mg/kg dry weight (bulk soil concentration of about 2650 mg/kg dry weight), and its transport to the aerial parts was extremely limited and was absent in the fruit. Cr showed a different trend compared to Pb with a concentration in the order of tens of mg/kg dry weight in the roots (bulk soil concentration ranging from 53.1 (PR) to 119.3 (PG) mg/kg dry weight), with irrelevant concentrations in stems, leaves and fruit. On the contrary, Cd had a higher ability to transport from roots to aerial parts compared to Cr and Pb. However, it must be considered that the experimental design of this study was characterized by a strong contamination of the soil in order to generate perceptible changes in the plant PTE intake capacity and in its metabolism. Moreover, the BCF values revealed that none of the PTEs was able to bio-concentrate in tomato plant roots, showing BCF values lower than 1. However, the TF values strongly suggest that among the three PTEs, only Cd showed partial ability to translocate into the above- ground parts of the plants. Additionally, the Pomodoro Giallo plants showed a stronger ability of translocation of Cd compared to San Marzano Cirio 3 plants, with TF values higher than 1 even at the lowest percentage (5%) of Cd. These results are confirmed by Trebolazabala and co-authors [ 38 ], who assessed the concentration of some potentially toxic elements, including Cd, Pb and Cr, in soils and in the different parts of 11 tomato cultivars, grown in Biscay, in Northern Spain. These authors observed that the concentrations of such PTEs were significantly higher in the soil than in the plants and that PTEs were accumulated in roots and leaves but not in the fruit. Similarly, in the study of Opeolu et al. [ 39 ], Pb accumulated in the roots, but not in the fruit. On the contrary, in a recent study, Magaji and collaborators [ 40 ] detected heavy metals (Cd, Cu, Pb and Zn in mg/kg) in vegetables obtained from Kembu farms and observed that only Pb exceeded the permissible limit of 0.3 mg/kg in tomatoes. Finally, in accordance with the results of the present study, Gharaibeh and collaborators [ 41 ] showed that tomato plants, grown in the presence of Cd, had a higher concentration in the roots compared to the fruit. In another study, Raptis and co-authors [ 42 ] showed that the uptake of Cr in lettuce shoots and roots increased in line with concentration of Cr in irrigation water. However, in the presence of high PTE concentration in the soil, most plants tend to limit the intake in the aerial parts and store toxic elements in the roots as they are not required for vegetal growth [ 15 ]. In addition, tomatoes show the potential to produce a higher content of secondary metabolites to protect themselves from toxic effects resulting from exposure to PTEs [ 14 ].
The results obtained from the PTEs at 10% of CEC of the soil suggest that the antioxidant activities of the tomato fruits can be limited or stimulated. The exposure to Cd at this level determined an increase of the antiradical scavenging activity (DPPH assay), in the methanolic and chloroformic extracts of PG fruits. In PG plants grown in uncontaminated soil, fruits were characterized by methanolic extract rich in polyphenols (474 mmol quercitin equivalents/kg of fresh products), and hydroxycinnamic acids such as caffeic acid and by the chloroformic extract rich in carotene as reported by Piscitelli et al. [ 6 ]. The exposure to Cr at the same level of Cd (10% of CEC) determined a significant increase of the antiradical scavenging activity (DPPH assay) in the hydrophilic extract of PG which is rich in polar phenolic compounds such as rutin and chlorogenic acid, hydro-soluble vitamins such as ascorbic acid. Differently from PG, PR did not show increase in radical scavenging properties with significant reduction in ABTS scavenging activity of methanolic extracts for each PTE. Therefore, it is most likely that the variety of tomato affects the tolerance to Cd, Cr and Pb. For example, some studies report high levels of antioxidant-producing enzymes (superoxide dismutase, ascorbate peroxidase and catalase) of tomatoes contaminated by heavy metals [ 43 – 45 ], contributing to the increment of biological properties in tomato fruits. These biochemical changes which increase the antiradical scavenging performance in the case of PG may be due to a possible hormetic effect, a stimulatory response to a certain level of soil contamination and inhibitory effect at higher levels. Indeed, in the present study, for higher levels of PTEs (20% of CEC) the plants did not bear fruits or died. The effects observed in fruit of the plants grown at the highest level of Cr and Cd were likely caused by their potential ability to generate oxygen species which may cause lipid peroxidation, reduction of photosynthetic activity and of chlorophyll biosynthesis, reduction of growth or alteration of water balance [ 31 ]. This oxidative stress may decrease adaptive responses of plants to metal ion homeostasis and redox balance precluding the switch in growth/defence equilibrium.
Conclusions
Differently from Cr and Pb which did not confirm translocation in the edible part of the plants, Cd was able to reach the fruits albeit at very low concentrations, significantly lower than those established by the regulation for fresh fruits. Despite Cd translocation, phenotypical effects were not observed up to a contamination level of 10% of CEC while significant differences in the antiradical properties were found for each PTE and for each cultivar. Types, concentrations and activities of PTEs modulated antiradical mechanisms with stimulatory or inhibitory responses, which most likely depended on the content of secondary metabolites produced by tomato plants in an effort of self-protection against the exposure to such elements. This study highlighted that, despite the experimental higher concentrations of PTEs compared to those occurring in the Sarno basin, strongly contaminated by the PTEs herein analyzed, the translocation of the elements to the edible part was rather low or absent. The potential health risk due to the vegetable consumption of crops grown in soils contaminated at very lower concentrations, in any case it is not enhanced also because of the ability of the tomato plants to reduce the PTEs transfer from the soil to the fruits. However, further studies from genetic-molecular to physiological and ecological levels are required for a better understanding of defensive/adaptive response mechanisms resulting from tomato plants exposed to toxic elements. Furthermore, the contribution of vegetable species consumption may be further developed to acknowledge potential health risks for residents in contaminated areas especially in a vulnerable population, such as children.
Supporting information
S1 fig. pomodoro giallo plants grown in experimental contaminated soils..
Pomodoro Giallo plants, grown in experimental soils contaminated with Cd, Cr and Pb at increasing levels (5, 10 and 20%) in relation to soil CEC values. From the top to the bottom, untreated tomato plants (NC = Negative Control) and plants treated with Cd, Cr and Pb.
https://doi.org/10.1371/journal.pone.0237031.s001
S2 Fig. San Marzano Cirio 3 plants grown in experimental contaminated soils.
San Marzano Cirio 3 plants, grown in experimental soils contaminated with Cd, Cr and Pb at increasing levels (5, 10 and 20%) in relation to soil CEC values. From the top to the bottom, untreated tomato plants (CN = Negative Control) and plants treated with Cd, Cr and Pb.
https://doi.org/10.1371/journal.pone.0237031.s002
Acknowledgments
The authors are thankful to Prof. Giuseppina Caraglia -native English speaker- (Dipartimento di Scienze e Tecnologie Ambientali, Biologiche e Farmaceutiche, Università della Campania “Luigi Vanvitelli”) for the English editing of the manuscript.
- View Article
- PubMed/NCBI
- Google Scholar
- 7. ISTAT—Istituto nazionale di statistica 2019. dati.istat.it/DCSP_COLTIVAZIONI
- 13. Kabata-Pendias A. Trace elements in soils and plants. 4th edition. CRC Press Taylor& Francis Group 2011.
- 18. Ministero delle Politiche Agricole e Forestali (Mi.P.A.F.). Determinazione del contenuto di cadmio, cobalto, cromo, rame, manganese, nichel, piombo, zinco estraibili in acqua regia. In: Angeli, F. Metodi di Analisi Chimica dei Suoli, Milano. 2000.
- Tools and Resources
- Customer Services
- Agriculture and the Environment
- Case Studies
- Chemistry and Toxicology
- Environment and Human Health
- Environmental Biology
- Environmental Economics
- Environmental Engineering
- Environmental Ethics and Philosophy
- Environmental History
- Environmental Issues and Problems
- Environmental Processes and Systems
- Environmental Sociology and Psychology
- Environments
- Framing Concepts in Environmental Science
- Management and Planning
- Policy, Governance, and Law
- Quantitative Analysis and Tools
- Sustainability and Solutions
- Share This Facebook LinkedIn Twitter
Article contents
Tomatoes: a model crop of solanaceous plants.
- Raheel Anwar , Raheel Anwar University of Agriculture Faisalabad
- Tahira Fatima Tahira Fatima Purdue University, Department of Horticulture and Landscape Architecture
- and Autar K. Mattoo Autar K. Mattoo United States Department of Agriculture
- https://doi.org/10.1093/acrefore/9780199389414.013.223
- Published online: 30 October 2019
The modern-day cultivated and highly consumed tomato has come a long way from its ancestor(s), which were in the wild and not palatable. Breeding strategies made the difference in making desirable food, including tomato, available for human consumption. However, like other horticultural produce, the shelf life of tomato is short, which results in losses that can reach almost 50% of the produce, more so in developing countries than in countries with advanced technologies and better infrastructure. Food security concerns are real, especially taking into consideration that the population explosion anticipated by 2050 will require more food production and the production of more nutritious food, which applies as much to the tomato crop as the other crops. Today’s consumer has become aware and is looking for nutritious foods for a healthful and long life. Little was done until recently to generate nutritionally enhanced produce including fruits/vegetables. Also, extreme environments add to plant stress and impact yield and nutritional quality of produce. Recent developments in understandings of the plant/fruit genetics and progress made in developing genetic engineering technologies, including the use of CRISPR-Cas9, raise hopes that a better tomato with a high dose of nutrition and longer-lasting quality will become a reality.
- fruit ripening
- genetic engineering
- abiotic stress
- biotic stress
Introduction
The tomato has become an important vegetable/fruit of high economic value worldwide due to its status as a high-yielding (37.6 tons/hectare) crop among other commonly consumed vegetables (Table 1 ), with its tasty fruit and richer dose of healthful nutrients, and particularly its carotenoid/lycopene content (Table 2 ) and it amenability as a homegrown (kitchen/home gardening) vegetable. Among the top 10 vegetables produced in the world, tomato ranks at the top and is one of the highest traded commodities (Table 1 ). Fresh tomatoes are consumed in salads. Minimally processed tomatoes are available in canned or crushed forms, whereas fully processed tomatoes include juice, ketchup, paste, purée, and sauce. In most countries, tomato constitutes a major food component of the daily diet.
Major tomato-cultivating countries that are responsible for 61% of the world’s tomato resources include China (33%), India (11%), Turkey (7%), the United States (6%), and Egypt (4%) (Figure 1 ). Other high-producing countries are Iran, Italy, Spain, Mexico, Brazil, Nigeria, and Russia. In 2016 , the global export of fresh and processed tomatoes generated US$12.8 billion in revenues, while by 2017 total tomato production in the world reached 182.3 million tons from 4.8 million hectares. Mexico, the Netherlands, and Spain were the top three tomato-exporting countries, with an export share of 22%, 13%, and 12%, respectively (Figure 2 ). Other major exporting countries of fresh tomato include Morocco, Turkey, Jordan, India, France, Belgium, and the United States. Among these, the United States is not only the 10th-ranked tomato-exporting country but also ranked among the top fresh tomato-importing countries (Figure 2 ). Norway, Finland, Switzerland, Ireland, Sweden, Germany, Denmark, Austria, and Canada were among the top 10 importing countries in 2016 ; the imported fresh tomato costs them from US$2,475 to 1,611 per ton ( 2016 trade statistics, Figure 3 ). Thus, the tomato is an economically important crop the world over. Its demand has also created a need to produce newer, high-yielding varieties with better nutritional quality.
Figure 1. World tomato production map with percentage contribution of each country.
Figure 2. World tomato exporting and importing countries with percentage contribution of each country in trade volume.
Figure 3. World importing countries paying top price per ton. Only countries importing more than 10,000 tons are included.
The wild tomato plant originated from the warmer areas of South America, including Ecuador, Chile, and the Galapagos Islands (Peralta & Spooner, 2007 ; Robertson & Labate, 2007 ; Rothan, Diouf, & Causse, 2018 ), with Peru as its center of origin. Based on its physical characteristics, the tomato was placed alongside the potato in the genus Solanum (Linnaeus, in 1753 ); however, Philip Miller placed it in a new genus and called it Lycopersicon esculentum . Lycopersicon esculentum Mill. is considered as a progenitor of large-fruited cultigens (Bailey, 1896 ; Robertson & Labate, 2007 ). Molecular phylogeny resolved the taxonomic status and placed tomato back under the genus Solanum , viz., Solanum lycopersicum L. (Peralta, Knapp, & Spooner, 2006 ). Cultivation, selection, and domestication of tomato species have been practiced since the dawn of civilization.
The tomato as a perishable horticultural crop has become an established model system for studying the intricate nuances of fleshy fruit development, including the ripening, postharvest, and senescence stages. It is sensitive to temperatures below 10ºC (leading to chilling injury) and above 28ºC (leading to heat stress), which cause damage. This precludes the use of cold storage for longer periods as well as long-distance transportation during hot summers, causing serious losses of tomato. Such postharvest losses create an obstacle to food security and economic losses during storage and long-distance shipping/transportation. The science of postharvest physiology and biochemistry of fruits/vegetables, including tomato, has transitioned in recent years with the advent of advanced molecular and biochemical approaches.
Table 1. Nutrient Facts of Fresh Tomato
*. Amounts per 100g Fresh Weight
Note . No amount of selenium, folic acid, vitamin B-12, retinol, β -cryptoxanthin, Vitamin D, cholesterol, ethyl alcohol, caffeine, or theobromine could be detected in fresh tomatoes (USDA, 2019 ).
Source : USDA ( 2019 ).
Table 2. Production, Area, and Yield of Top 10 Vegetables in the World
Source : FAO ( 2017 ).
These developments in understanding the players involved in tomato (fruit) biology and senescence can contribute to strategies for improving production, curtailing the losses due to short postharvest life and eventually the ability to manipulate the ripening process. A major hormone that regulates fruit ripening is ethylene, also called the “ripening hormone.” More is now understood about how ethylene induces ripening, its signal transduction pathway, and its interaction with other plant hormones in the process (Anwar, Mattoo, & Handa, 2019 ; Fatima et al., 2008 ; Harpaz-Saad, Yoon, Mattoo, & Kieber, 2012 ; Klee & Giovannoni, 2011 ; Mattoo & Handa, 2017 ; Nath, Bouzayen, Mattoo, & Pech, 2014 ). Advanced biotechnology and bioinformatic tools have helped to mine the data from studies involving genetically engineered lines and natural mutants of tomato, and the information generated has added to current understandings of the players that regulate tomato fruit ripening. Thus plant hormones, various transcription factors, small metabolites, micro RNAs (miRNAs), epigenetic factors, and other factors interactively regulate tomato fruit ripening (Anwar et al., 2019 ; Bapat et al., 2010 ; Bouzayen, Latche, Nath, & Pech, 2010 ; Handa, Anwar, & Mattoo, 2014 ; Klee & Giovannoni, 2011 ; Osorio & Fernie, 2013 ; Osorio, Scossa, & Fernie, 2013 ; Seymour, Chapman, Chew, & Rose, 2013 ; Seymour, Poole, Giovannoni, & Tucker, 2013 ). Scientists now have new toolsets available for improving the quality, flavor, taste, and shelf life of tomato. On the other hand, it has also become apparent that developing a tomato germplasm that is resistant to a variety of biotic and abiotic stresses is not easily achievable using simple and normal breeding strategies. This is so because these traits are not singular and are rather more complex than previously thought.
There is a need not only for developing tomato lines with higher fruit yield, longer shelf life, and greater resistance to biotic and abiotic stressors but also for enhancing the levels of health-promoting nutrients (Causse, Giovannoni, Bouzayen, & Zouine, 2016 ; Kaur, Handa, & Mattoo, 2017 ). The educated consumers’ demand for nutritionally enriched fruits and vegetables has intensified, particularly for tomatoes. However, these nutrients are often present in tomato fruit at only marginal concentrations for human nutrition/prevention of disease. The nutrient load is primarily dependent on crop genetics and is regulated by complex developmental and environmental cues (Roberts & Mattoo, 2019 ). Vitamin C (ascorbic acid) is an essential nutrient in the human diet (Mellidou & Kanellis, 2017 ). Green tomato fruits contain relatively high amounts of total ascorbic acid (23.4 mg per 100 g), which decreases to 13.7 mg per 100 g in red ripe tomatoes (USDA, 2019 ) (Figure 4 ).

Figure 4. Growth habit of tomato plants (i.e., indeterminate plants [left] and determinate plants [right]). Indeterminate tomato plants are generally grown under structures where vines can be trained on hanging strings while determinate plants are low statured and can be grown in open fields.
Although commercially cultivated tomato varieties ( Solanum lycopersicum accessions) are considered a good dietary source of ascorbic acid, tomato’s wild progenitors such as Solanum pennellii have much higher ascorbic acid content (47.5 mg per 100 g) (Di Matteo et al., 2010 ). This means that ascorbic acid gradually declined during selection and domestication of tomato genotypes. On the other hand, phytonutrient lycopene, an important carotenoid that provides red pigmentation of ripe tomato fruit, is known for its antioxidant/anticancer properties (Giovannucci et al., 2002 ). However, its levels are not sufficient for a good dietary dose. Lycopene-enriched and longer shelf-life tomato fruit (Mehta et al., 2002 ) and anthocyanin-rich tomatoes (Butelli et al., 2008 ) have been genetically engineered. However, more needs to be done to provide the consumer with a tastier tomato enriched with a high dose of healthful compounds for a healthy life.
Agronomic Aspects of Tomato Production
Tomato is a commonly grown vegetable in most countries, and the production systems employed include open field, tunnels, net house, greenhouses, and soilless culture, like hydroponics. Its growth habit can vary to be determinate or indeterminate (Figure 5 ).
Figure 5. Endogenous levels of ascorbic acid during tomato fruit maturation.
Determinate tomatoes flower almost at the same time, contain a predetermined number of clusters, and grow to small heights. Indeterminate tomato plants keep growing and produce flower clusters until the weather prohibits (tomato plants are sensitive to cooler temperatures such as below 10º–15ºC). Indeterminate plants need more plant care than determinate ones; the older leaves on the lower part of the growing stem need to be excised, while the continuously elongating stems need support with strings so that they do not droop and fall to the ground. Indeterminate varieties have higher yields than the determinate types. Indeterminate varieties are generally managed on strings under tunnels or in a greenhouse, whereas determinate tomato plants are usually grown in open fields.
Tomato is a warm season crop. Therefore, during the off-season (cooler temperatures), farmers the world over have the opportunity to invest in a tunnel system for off-season production of tomatoes. Such a production business has gained popularity. Off-season harvest generally hits the market when open field production has yet to arrive. Tomato plants grown under protective structures are generally uniform in size and quality and give consistent yield for an extended period. Because tomato crops are sensitive to frost injury, transplantation of tomato seedlings in the open field is started only after the frost spell. They are mostly grown in loamy soils during the dry season. During early growth, proper weed management and moist soil around seedlings favors a quick crop stand. A good mulch (preferably legume based) under the plant canopy enables the soil to remain moist and deters weed growth. In mechanized countries, tomatoes are grown in open field, harvested mechanically all at once, and then transported in bulk, whereas indeterminate varieties are generally preferred for fresh market tomatoes as these are juicier. Since indeterminate varieties keep producing flowers and fruits, they require multiple picking, usually by hand. Due to the demand for indeterminate varieties, farmers have started growing indeterminate tomato plants in open fields using various support systems such as strings or wire nets.
From Flowering to Fruit Development to Ripening
Flowering is a genetically programmed event in angiosperms in which seeds are enclosed in an ovary. Factors such as endogenous molecules, environmental cues, production practices, and/or external stressors can alter the timing and intensity of the floral transition. Since the time of tomato domestication, genetic mutations in growth factors that control the flowering in response to internal and external cues led to the expansion of tomatoes beyond their native geographical locations. Today more is understood about the key elements and genetic players that impact the life of a plant from vegetative growth to flowering to fruit development to fruit ripening, due largely to advances in biotechnology and genetics. Progress toward developing toolsets of engineered genes and metabolic pathways have contributed to current understandings about plant life at large and plant processes in general. For example, plant switching from the vegetative to the reproductive phase involves floral transition of the shoot apical meristem and development of inflorescence architecture, which are controlled by regulatory interactions of several genetic factors (Huang et al., 2018 ; Périlleux, Lobet, & Tocquin, 2014 ; Silva Ferreira et al., 2018 ; Xu, Park, Van Eck, & Lippman, 2016 ). Identification of transcription factors that have specific binding sites on cognate gene(s) has added to knowledge of how genes are regulated. For example, transcription factor AGAMOUS-LIKE1 (TAGL1) regulates sepal and carpel size (Vrebalov et al., 2009 ). Thus an florigen paralog and flowering repressor SELF PRUNING 5G ( SP5G ), not expressed in cultivated varieties, was found to be highly expressed in the long-day wild tomato. Meanwhile, a scientific breakthrough led to a new genome editing toolset called CRISPR/Cas9, which can change gene function by altering DNA sequence in a highly specific manner (reviewed in Lander, 2016 ). Utilizing the CRISP/Cas9 technology to silence the SlSP5G gene in tomato led to more compact growth, initiation of early flowering, and earlier harvesting of the engineered tomato fruit (Soyk et al., 2016 ).
Fruit development is the terminal stage in plant ontogeny. Selection and domestication of the wild small fruiting tomato ancestor Solanum pimpnellifolium that led to present-day commercially grown tomato cultivars also brought about evolution of diversity in tomato fruit shape and size. Utilizing this genetic diversity enabled identification and characterization of two genes regulating variation in weight ( FW2.2 , FW3.2 ) and four genes ( SUN , OVATE , LC , FAS ) that regulate the shape of tomato fruit (van der Knaap et al., 2014 , and references therein). Glucose and hormones, mainly auxin, gibberellins, and cytokinin, are vital for fruit set (Kumar, Khurana, & Sharma, 2014 ; Liu et al., 2018 ; Ruan, Patrick, Bouzayen, Osorio, & Fernie, 2012 ). Hormonal signaling and carbon partitioning also tightly control rapid division and expansion of cells as well as dramatic shifts in metabolic profiles in expanding fruits. This phase is also marked by the accumulation of phenolic compounds, soluble solids, organic acids, and anthocyanins (Dardick & Callahan, 2014 ; Handa, Tiznado-Hernández, & Mattoo, 2012 ; Hiwasa-Tanase & Ezura, 2014 ; Klie et al., 2014 ; McAtee, Karim, Schaffer, & David, 2013 ; Seymour, Østergaard, Chapman, Knapp, & Martin, 2013 ). A blend of these metabolites in edible tissues of tomato (epicarp, septum, and interlocular tissues) can determine nutritional and sensory quality of a red ripe tomato. From a consumer perspective, sweetness, glutamic acid, titratable acidity, and juiciness are major quality benchmarks (Casals, Rivera, Sabaté, Romero del Castillo, & Simó, 2018 ), in addition to health-promoting benefits of several nutrients present in tomato.
The final phase of tomato plant development leads to a red ripe fruit, and the photosynthesizing organelle chloroplast transitions into an organelle called chromoplast, in which human health promoting carotenoids including lycopene and β -carotene accumulate. Lycopene forms 70% to 90% of total carotenoids present in tomato fruit and, as stated earlier, is the reason for the red color of ripe tomato fruit, whereas β -carotene content forms 5% to 40% of total carotenoids contributing the orange color of tomatoes (Burns, Fraser, & Bramley, 2003 ; Ronen, Carmel-Goren, Zamir, & Hirschberg, 2000 ). Phytochrome pigment is involved in photomorphogenesis, and phytochrome-interacting factors trigger biosynthesis of carotenoids—lycopene, carotenes, zeaxanthin, and lutein—as well as the carotenoid-derived volatiles (Fantini, Falcone, Frusciante, Giliberto, & Giuliano, 2013 ; Llorente, D’Andrea, & Rodríguez-Concepción, 2016 ; Seymour, Østergaard, et al., 2013 ; Tomato Genome Consortium, 2012 ). During tomato fruit maturation, the carotenoid synthesis enzyme phytoene synthase 1 ( SlPSY1 ) is inhibited via direct interaction with the STAY-GREEN protein ( SlSGR1 ) to regulate chlorophyll degradation in tomato leaves and fruit. In this colors-changing scheme, one other master fruit-ripening regulator called SlMADS-RIN controls PSY function (Fujisawa, Nakano, Shima, & Ito, 2013 ). Thus the tomato fruit undergoes changes in pigmentation upon ripening from a green (chlorophyll intense) and solid mature fruit to red (or orange) (chromoplast intense) fruit. β -carotene is utilized in the synthesis of vitamin A and another hormone called abscisic acid (ABA). The life of a transforming and transitioning tomato fruit from green to red is coordinated by interactions of various but specific gene players, including small heat shock protein ( HSP21 ) (Neta-Sharir, Isaacson, Lurie, & Weiss, 2005 ), and may involve light (via reduction in the expression of ETHYLENE RESPONSE FACTOR E4 [ SlERFE4 ]) and upregulation of both AUXIN RESPONSE FACTOR 2 paralogues ( SlARF2a / b ) (Cruz et al., 2018 ).
Ethylene: A Fruit-Ripening Hormone
The dissipation in green color at the onset of the tomato fruit ripening phase is associated with a climacteric burst in respiration (involving the enzyme alternate oxidase) and ethylene production (Cherian, Figueroa, & Nair, 2014 ), transformation of chloroplasts into chromoplasts, and loss of photosynthetic membrane integrity (Yazdani et al., 2019 ). Ethylene is a gaseous hormone fundamental to the fruit ripening process in many fruits, including tomato. In addition to ethylene, other developmental factors also contribute to tomato fruit ripening. Fruit characteristics are irreversibly altered due to pigmentation and alteration in volatile and bioactive compounds (carotenoids, anthocyanin, flavonoids, volatiles, lipids, etc.) and changes in cell wall integrity and cellular constituents (Bouzayen et al., 2010 ; Handa et al., 2014 ; Handa, Srivastava, et al., 2010 ; Klee & Giovannoni, 2011 ; Osorio et al., 2012 ; Osorio et al., 2013 ; Valero & Valero, 2013 ). Dynamic morphological and biochemical changes in a ripening fruit are spatiotemporally regulated by various transcription factors, signaling molecules/hormones and their crosstalk at genetic and epigenetic levels (Giovannoni, Nguyen, Ampofo, Zhong, & Fei, 2017 ).
Ethylene production during plant growth and development has two distinct phases called “system 1” and “system 2.” Ethylene production in vegetative tissues and unripe fruits is regulated in an autoinhibitory manner, which maintains its basal level; this mode of ethylene synthesis is system 1. On the other hand, ethylene is produced at higher levels during flower senescence and fruit ripening; this mode of ethylene synthesis is autocatalytic and known as system 2. Climacteric fruits, such as tomato, exhibit a simultaneous burst in ethylene production, which is its major role as a fruit-ripening hormone. Intricate details about production, perception, and mode of action of ethylene and what regulates fruit ripening are now known. The enzyme 1-aminocyclopropane-1-carboxylate synthase (ACC synthase) is critical for the synthesis of 1-aminocyclopropane-1-carboxylate (ACC), which is the immediate precursor of ethylene. ACC is oxidized by the terminal enzyme called ACC oxidase (ACO) to produce ethylene. The importance of these two molecules in ethylene biology is apparent from the fact that at least nine ACS (ACS1A, ACS1B, ACS2-8), five ACO (ACO1-5), six ethylene receptors ( ETR1 , ETR2 , ETR4 , ETR5 , ETR6 , ETR3 / Never-ripe ), and four EIN3-like (EIL) genes have been identified that orchestrate ethylene signaling cascade (see Paul, Pandey, & Srivastava, 2012 , and references therein). A global view of transcriptional network and temporal changes of ethylene-responsive genes in tomato fruit development/ripening is also available (Liu, Yu, et al., 2015 ; Sharma et al., 2010 ). Ethylene also coordinates with other ripening-related developmental factors, namely, auxin, ABA, RIN, NON-RIPENING (NOR), CNR, and TAGL1, to regulate different processes during plant and fruit development (Mou et al., 2016 ; Osorio et al., 2011 ). Three types of transcriptional feedback circuits proposed to control ethylene-dependent ripening in climacteric fruits such as tomato are MADS-type circuit, peach NAC-type circuit, and banana dual-loop circuit (Lü et al., 2018 ). 1 H-nuclear magnetic resonance spectroscopy was employed to dissect metabolic pathways that are ethylene-dependent or ethylene-independent in unique “transgenic” tomato lines, one with impaired expression of ACC synthase-2 gene and the other a progeny of its genetic cross with a line overexpressing yeast S -adenosylmethionine decarboxylase (Sobolev et al., 2014 ). It enabled the identification of ethylene-dependent metabolites (increase in amino acids glutamate and aspartate plus adenosine monophosphate [AMP]) and those that were ethylene-independent (alanine, tyrosine, valine, isoleucine, phenylalanine, malic acid, and myo-inositol). Such approaches are important for modifying metabolic pathways to either add a dose of nutrients to a produce (tomato fruit) or reduce unwanted or antinutrition molecules.
Hormones Abscisic Acid, Auxin, and Polyamines Have a Stake With Ethylene in Fruit Ripening
In addition to ethylene, the hormone ABA has a say in controlling ripening in tomato since the climacteric burst of ethylene was found to be accompanied by a sharp increase in ABA content (Leng, Yuan, & Guo, 2014 ). Exogenous ABA application promoted not only the expression of ethylene regulators ( RIN , TAGL1 , CNR , NOR ) but also those involved in ethylene production and response ( ACS4 , ACO1 , GR and ETR6 ) (Mou et al., 2016 ; Zhang, Yuan, & Leng, 2009 ). Reciprocally, ethylene is required for the induction of ABA synthesis (Mou et al., 2016 ). Opposite to ethylene and ABA, another plant hormone, auxin (IAA), delays fruit ripening via impact on ripening regulators (RIN, ethylene, and ABA) and biosynthesis of lycopene and β -xanthophyll (Su et al., 2015 ). Out of 25 identified Aux/IAA genes in tomato, five ( IAA3 , IAA4 , IAA9, IAA15 and IAA27 ) mingle with fruit ripening (Audran-Delalande et al., 2012 ). Light is a factor for carotenoid (nutrition) accumulation by repressing these Aux/IAA genes (Cruz et al., 2018 ). AUXIN RESPONSE FACTOR 4 ( ARF4 / DR12 ) was found to promote catabolism of starch into glucose and fructose pinpointing role of auxin in essential cellular processes in tomato fruit (Dai et al., 2016 ; Jones et al., 2002 ; Sagar et al., 2013 ).
Polyamines are ubiquitous natural plant growth regulators among which the most studied include putrescine, spermidine, spermine, and thermo-spermine (Mattoo, Fatima, Upadhyay, & Handa, 2014 ; Mattoo, Minocha, Minocha, & Handa, 2010 ). Using a genetic engineering approach enabled accumulation of spermidine and spermine levels at the cost of putrescine in transgenic tomato (Mehta et al., 2002 ). These tomatoes were not only much richer in properties such as the anticancer carotenoid lycopene but also had better firmness and high juice viscosity, attributes that reinforced the promotive role of spermidine/spermine in improving tomato nutrition and quality (Handa, Nambeesan, et al., 2010 ; Kolotilin et al., 2011 ; Mehta et al., 2002 ; Nambeesan et al., 2010 ; Srivastava et al., 2007 ). Cross-linkages of polyamines with other plant hormones were found to be prevalent based on collation of transcriptomic data from different tomato genotypes (Anwar, Mattoo, & Handa, 2015 ). Thus polyamine crosstalk with plant signaling hormone genes was unraveled. Spermidine was found to be negatively linked with ABA biosynthesis but positively linked with genes for the biosynthesis of hormones ethylene, jasmonate, and gibberellins. Also, spermidine and spermine were positively regulated with salicylic acid, auxin, and cytokinin signaling (Anwar et al., 2015 ). Metabolomic data obtained on these tomato fruits (engineered to have altered polyamine, ethylene, or methyl jasmonate levels) have paved the way for modulating tomato fruit attributes via a crosstalk between plant hormones ethylene/methyl jasmonate using sustainable cropping agroecosystems (Fatima et al., 2016 ).
Nitric oxide (NO) is another ubiquitous secondary messenger that, in response to environmental and endogenous cues, interacts with almost all plant hormones to varying degrees. NO is a negative regulator of ethylene (Manjunatha, Lokesh, & Neelwarne, 2010 ); in NO-fumigated tomatoes, ethylene production was inhibited, involving downregulation of transcription of ACC oxidase genes ( ACO1 , ACOH2 , ACO4 ) (Aboul-Soud, 2010 ; Eum, Kim, Choi, & Lee, 2009 ). NO S -nitrosylates methionine adenosyl transferase and reduces the availability of methyl substrates for ethylene biosynthesis (Manjunatha, Gupta, Lokesh, Mur, & Neelwarne, 2012 ). In addition to the reduction in transcription of ACC oxidase genes, sodium nitroprusside released NO can delay tomato pericarp reddening and enhance activities of enzymes such as superoxide dismutase, catalase, and peroxidase (Lai, Wang, Li, Qin, & Tian, 2011 ). Overaccumulation of NO in short root ( shr ) tomato mutant delayed fruit ripening and led to enhancement in tyrosine, indole-3-acetic acid, and indole-3-butyric acid but a reduction in fatty acids and ABA content (Bodanapu et al., 2016 ). NO negates ethylene perception in shr mutant fruits (Bodanapu et al., 2016 ). More information is required on the role of NO in delaying the ripening and extending the shelf life of tomato fruits.
Building Nutrition and Flavor-Rich Tomato Fruit for Promoting Human Health
Epidemiological studies support the common contention that foods rich in phytonutrients help in the maintenance and promotion of human health and reduce disease risk of certain cancers as well as cardiovascular and chronic degenerative diseases (Virtanen, Voutilainen, Nurmi, Tuomainen, & Mursu, 2013 ; Yamada et al., 2011 ). Examples of such phytonutrients include vitamins (tocopherols, tocotrienols, ascorbic acid, and dehydroascorbic acid), carotenoids, phenolics/flavonoids, triterpenoids, and glucosinolates, many of which have antioxidative and free radical scavenging properties (Gerster, 1997 ; Kanellis & Manganaris, 2014 ; Liu, 2013 ). The phytonutrient-based protective capacity against or ability to delay the incidence of fatal diseases is thought to involve their antioxidative and free radical scavenging properties, which protect cellular macromolecules from oxidative damage (Mattoo, Minocha, et al., 2010 ). Because disease is associated with highly oxidative milieu, the dietary phytonutrients have the potential of protecting against disease(s) (García-Mier, Guevara-González, Mondragón-Olguín, Del Rocío Verduzco-Cuellar, & Torres-Pacheco, 2013 ). Among these, lycopene is a carotenoid that has been linked to reduced risk of various cancers including of the prostate, lung, stomach, pancreas, cervix, and colon as well as colorectal, oral, and esophageal cancers (Agarwal & Rao, 2000 ; Giovannucci, 1999 ). The antioxidant capacity of lycopene in vitro is higher than other carotenoids, β -carotene, and vitamin E. Other than its antioxidant and anticarcinogenic properties, lycopene is cardioprotective, anti-inflammatory, and antimutagenic in nature (Friedman, 2013 ). Other phytochemicals present in tomato include phytofluene, polyphenols, and flavonoids (quercetin and kaempferol). The phenolic acids (polyphenols) and flavonoids also have antioxidant properties and have been associated with reduced risk of age-related diseases, heart ailments, and cancers (Ross & Kasum, 2002 ).
Given that fruits such as tomato contain healthful nutrients, the reality is that the levels of these phytonutrients in most produce is, in fact, below the recommended daily allowance for maintaining human health. The traditional/conventional plant breeding process has not been able to increase the endogenous levels of such phytonutrients because the endogenous metabolic pathways involved are highly regulated. Moreover, it takes 10 to 15 years to release a new tomato variety. There is thus an important need to enhance the endogenous levels of phytonutrients in vegetables and fruits, including tomato. Employing genetic engineering to substantially enhance the levels of phytonutrients in fruits crops, including tomato, is a good avenue, since this path has shown the promise of building robust phytonutrient levels in crop plants, including tomato (reviewed in Mattoo, Shukla, Fatima, Handa, & Yachha, 2010 ; see also Kaur et al., 2017 ).
Nutritional Carotenoids
Considerable experimentation has gone into enabling genetic manipulation of carotenoid content and elevating nutritional quality of tomato fruit (d’Ambrosio et al., 2004 ; Fraser et al., 2002 ; Guo, Zhou, Zhang, Xu, & Deng, 2012 ; Mehta et al., 2002 ; Nambeesan et al., 2010 ; Neily et al., 2011 ; Rosati et al., 2000 ). Engineering the levels of higher polyamines, spermidine and spermine, specifically in tomato fruit resulted in two- to threefold enrichment (120–175 µg/g fresh weight) in lycopene compared to the control line as well as the micronutrient choline in addition to the attribute of higher processing quality (Mattoo et al., 2006 ; Mehta et al., 2002 ). Further, manipulation of a bacterial gene (phytoene synthase [ crtB ]) in tomato fruit increased phytoene (2.4-fold), lycopene (1.8-fold), β -carotene (2.2-fold), and lutein levels (Fraser et al., 2002 ). Likewise, expression of the citrus lycopene β -cyclase ( crtl-b ) in tomato led to a fourfold increase in β -carotene levels (Guo et al., 2012 ). RNAi repression resulted in altered accumulation of phytoene and lycopene. This led to a fourfold increase in lycopene and a ninefold increase in β -carotene in red fruits of transgenic tomato (Luo et al., 2013 ). These positive findings have generated a lot of interest in manipulating carotenoid levels in tomato and, in the process, have helped enhance understanding of the means plants employ to regulate endogenous gene expression. Thus it has also become apparent that the plant hormone ABA is a negative regulator (Galpaz, Wang, Menda, Zamir, & Hirschberg, 2008 ; Sun et al., 2012 ) and an ORANGE (OR) gene a positive regulator of carotenoid synthesis (Yazdani et al., 2019 ) in tomato.
Flavonoids and Ascorbate (Vitamin C) Antioxidants
Other antioxidants that modulate tomato fruit color, flavor, and texture are called phenolic acids and flavonoids. Tomato fruit flesh has more carotenoids than flavonoids, raising a need also to enhance flavonoid content by genetic engineering (since this technology is more robust than known thus far). The main tomato flavonoids are naringenin-chalcone and rutin (quercetin rutinoside), which accumulate in the peel. Tomato fruits were engineered to constitutively overexpress petunia chalcone isomerase ( CHI ) and as much as 78-fold more flavonols (quercetin glycoside, rutin) accumulated in the fruit peel (Muir et al., 2001 ). Tomato fruit is deficient in making resveratrol because it lacks the gene stilbene synthase ( StSy ). This deficiency was circumvented by engineering heterologous plant genes and expressing them in tomato. This strategy enabled enrichment of flavonoids (D’Introno et al., 2009 ; Giovinazzo et al., 2005 ; Schijlen et al., 2006 ), trans -resveratrol, and trans -glucopyranosides (piceid) (D’Introno et al., 2009 ; Giovinazzo et al., 2005 ; Schijlen et al., 2006 ) in transgenic tomato fruit. Likewise, a combined overexpression of two flavonoid biosynthesis pathway genes, chalcone synthase ( CHS1 ) and chalcone reductase ( CHR ) from Petunia and Alfalfa, respectively, enabled threefold higher levels of novel flavonoids, flavones, and flavonols (butein, isoliquiritigenin, naringenin, chalcone, and rutin in tomato (Schijlen et al., 2006 ). Apart from achieving higher levels of flavonoids (flavones and flavonols), the total antioxidant capacity of tomato fruit peel was increased approximately threefold (Schijlen et al., 2006 ).
Transcription factors are regulators of gene expression, and their use has enabled engineering the levels of many other health-promoting molecules. For instance, tomato fruit-specific enrichment with anthocyanins was achieved by expressing snap dragon transcription factors (Rosea1 [ Ros1 ] and Delila [ Del ]) (Butelli et al., 2008 ). Property of anthocyanins lies in the protection against certain cancers, cardiovascular disease, and age-related degenerative diseases; in addition, anthocyanins are anti-inflammatory in nature and promote visual acuity as well as lessen the risk of obesity and diabetes. Transcriptional regulation of secondary metabolism was apparent from an earlier study in which maize transcription factors (MYB-type C1 and MYC-type LC) were used to transform tomato fruit for increasing the levels of higher flavonoids, mainly kaempferol content (Bovy et al., 2002 ). Notably, when Arabidopsis transcription factor AtMYB12 was employed to enhance the levels of flavonoids in tomato, it led to high amounts of chlorogenic acid (27-fold), dicaffeoyl quinic acid (26-fold), tricaffeoyl quinic acid (42-fold), quercetin rutinoside (67-fold), and kaempferol rutinoside (593-fold) in the transgenic tomato fruit (Luo et al., 2008 ). Following the same strategy, overexpression of a flavonoid-related R2R3-MYB transcription factor ANTHOCYANIN1 ( ANT1 ) from wild tomato ( Lycopersicon chilense ) enhanced anthocyanadins, including petunidin, malvidin, and delphinidin in the fruit (Schreiber et al., 2012 ). The strength of AtMYB12 in enhancing the content of different flavonoids was followed by another study that utilized this transcription factor to augment levels of flavonols and hydroxycinnamates by 10% (100 mg per g DW) of fruit dry weight, likely via increased supply of carbon from primary metabolism to secondary metabolism (Zhang et al., 2015 ). This latter point is important because it is the secondary metabolism, dependent on carbon supply from primary metabolism, that is basically what leads to the synthesis of many nutritionally important biomolecules.
Ascorbic acid (vitamin C) is another essential nutrient in the human diet and commonly found in tomato and other fruits/vegetables (Mellidou & Kanellis, 2017 ). It is important for tissue repair, immune system function, formation of collagen, and generation of neurotransmitters. It is an antioxidant important for maintaining healthy skin, cartilage, teeth, bone, and blood vessels. A deficiency of ascorbic acid results in development of scurvy, a disorder causing muscular weakness, rash, and joint pain. Ascorbate-rich fruits including tomato are essential for human health. Improving vitamin C content in tomato fruit is becoming a desirable goal of breeding programs. According to various national agencies, the recommended dietary allowance of ascorbic acid has been set anywhere from 45 mg per day (World Health Organization) to as much as 110 mg per day (European Food Safety Authority). In tomato fruits, total ascorbic acid actually decreases as the fruit matures (Figure 5 ). Green tomato fruits contain a relatively higher amount of total ascorbic acid (23.4 mg per 100 g), but these levels decrease to 13.7 mg per 100 g in red ripe tomatoes (USDA, 2019 ). The wild progenitor Solanum pennellii of commercially cultivated tomato varieties ( Solanum lycopersicum accessions) is much richer in ascorbic acid content (47.5 mg per 100 g) (Di Matteo et al., 2010 ), which means that ascorbic acid levels gradually declined with the advancement in selection and domestication of tomato genotypes. Ascorbic acid pool size is mainly governed by two critical enzymes. GDP- D -mannose-3,5-epimerase (GME) converts GDP- D -mannose into GDP- L -galactose (Bulley & Laing, 2016 ) whereas GDP- L -galactose phosphorylase (GGP) catalyzes the conversion of GDP-L-galactose to L-galactose-1-P (Mellidou & Kanellis, 2017 ). Expression studies have confirmed a positive correlation between transcript levels of genes encoding these enzymes with total ascorbic acid concentration during tomato fruit ripening (Ioannidi et al., 2009 ; Mellidou et al., 2012 ; Wang et al., 2013 ), suggesting a strong influence of GDP- D -mannose-3,5-epimerase and GDP- L -galactose phosphorylase on ascorbic acid biosynthesis.
Fruit Aroma and Flavor Volatiles
Volatile compounds contribute to aroma and flavor of the fruit (Baldwin, Kessler, & Halitschke, 2002 ). Major aromatic compounds include esters, ketones, terpenoids, lactone, aldehydes, and alcohols. In tomato, 20 to 30 aromatic volatiles are present in quantities sufficient enough to stimulate human olfactory system and establish tomato flavor (Klee & Giovannoni, 2011 ). Hexanal, Z -3-hexenal, E -2-hexenal, hexanol, and Z -3-hexenol are major volatile compounds derived from oxygenation of unsaturated fatty acids (Bai et al., 2011 ). Although extensive studies in the past have added a wealth of information about aromatic pathways in tomato fruit and the contribution of major volatile compounds, relatively little has been accomplished in the last decade. However, overexpression of aromatic L -amino acid decarboxylases ( LeAADC1A and LeAADC2 ) in tomato led to a 10-fold increase in the emission of volatiles, 2-phenylacetaldehyde and 2-phenylethanol (Tieman et al., 2006 ). TomloxC is one of the tomato lipoxygenase family of genes (Upadhyay & Mattoo, 2018 ), which is involved in generating fatty acid-derived flavor components in tomato (Chen et al., 2004 ). Reduction in TomloxC transcripts via a transgenic strategy caused reduction in the flavor volatiles including hexenal, hexanal, and hexanol, data that helped to link this gene with the aroma/flavor attributes in tomato (Chen et al., 2004 ). An academic offshoot of experimenting to enhance fruit flavor and volatiles has been the discovery of rigid regulation of secondary metabolism in plants, including tomato. Thus an increase in phenylpropanoids was not followed by an increase in phenylalanine-derived flavor volatiles, suggesting the existence of other factors that limit synthesis of flavor volatiles even when the substrate is available (Cin et al., 2011 ). Tomato genotypes containing loci from wild species Solanum pennellii thereafter revealed that a complexed molecule, 4-coumarate:CoA, redirects the flux of phenylpropanoids toward biosynthesis of glycosides in tomato fruit (Rigano et al., 2016 ).
In the context of developing a flavorful tomato to meet the consumer demand for a tasty product, a major study has revealed that traits defining volatile molecules have been randomly lost due to domestication of tomato during breeding strategies for producing a large size tomato (Tieman et al., 2017 ). It is apparent that the transgenic approach to enhance the potential of edible produce is a valuable approach, particularly to enhance flavor and quality of tomato for human health. One consequence of engineering tomato fruit with a higher level of growth regulators such as spermidine and spermine has been significantly higher fructose/glucose and acid [citrate+malate]/sugar [glucose+fructose+sucrose] ratio in the engineered red fruits (Mattoo et al., 2006 ), an attribute considered as higher quality in tomato breeding programs. Other examples include enhancing the contents of vitamin C (Garchery & Gest, 2013 ; Garcia et al., 2009 ; Zhang et al., 2011 ) and folates (de la Garza et al., 2007 ; Waller et al., 2010 ).
Epigenetics: Additional Check Point for Improving Tomato Fruit
Epigenetics involves switching genes on or off via DNA methylation/demethylation process and regulates how cells regulate gene function without changing the DNA sequence. Thus, such combinatorial gene mapping can help to change a gene’s state and aid toward engineering plants by removing/silencing unwanted genes and keeping good ones intact and active. One can then develop new plants whose fruit such as tomato can acquire and maintain a high dose of nutrition, longer-lasting quality, and improved taste/flavor. Epigenetics can thus influence how cells produce relevant proteins, thereby it adds another layer over coordinated development programs, for example, fruit maturation and ripening (Farinati, Rasori, Varotto, & Bonghi, 2017 ). The role of DNA methylation in the regulation of fruit ripening in tomato has begun to emerge. During fruit development, DNA methyltransferase 1 ( MET1 ), chromomethylases, and domains rearranged methyltransferases keep ripening genes methylated by adding a methyl group to carbon 5 of cytosine (5-methylctyosine: 5mC), thus silencing developmentally regulated CHROMOMETHYLASE3 ( SlCMT3 ) reduced methylation at CHG sites in the promoter of SBP-box transcription factor COLOURLESS NON-RIPENING ( CNR ) and in the genomic regions associated with MADS-RIN binding sites (Chen et al., 2015 ). Promoters of ripening-related genes in ripening-impaired mutants ( Cnr and rin ) are consistently methylated as compared to a gradual decline of methylation levels observed in wild-type fruits (Zhong et al., 2013 ). When tomato plants were exposed to methyltransferase inhibitor 5-azacytidine, it causes demethylation of RIN-binding sites and results in premature ripening of fruit (Zhong et al., 2013 ). Eight genes putatively encoding 5mC methyltransferases and four DEMETER-LIKE DNA demethylases have been identified in tomato (Chen et al., 2015 ; Teyssier et al., 2008 ).
These demethylases remove the epi-marks leading to the ripening processes (Gallusci, Hodgman, Teyssier, & Seymour, 2016 ; Law & Jacobsen, 2010 ; Zhu, 2009 ). In tomato, RNAi-mediated suppression of SlDML2 resulted in hyper-methylation and transcriptional repression of RIN , NON-RIPENING ( NOR ), and carotenoid synthesis gene, PHYTOENE SYNTHASE 1 ( PSY1 ) (Liu, How-Kit, et al., 2015 ). Reduction in the transcript levels of SlDML2 in the ripening mutants of tomato (Liu, How-Kit, et al., 2015 ), along with a strong expression of SlDML2 and SlDRM7 during early phases of fruit ripening (Liu, How-Kit, et al., 2015 ; Teyssier et al., 2008 ), are suggestive of the existence of a regulatory loop between DNA demethylation machinery and RIN, NOR, and CNR transcription factors during tomato fruit ripening (Gallusci et al., 2016 ; Liu, How-Kit, et al., 2015 ). Reciprocally, an interplay of methylome dynamics with similar transcription factor is also possible (Giovannoni et al., 2017 ).
SlDML2 -mediated demethylation has been shown to induce transcription of genes involved in the synthesis and signaling of ethylene, production of pigments/flavor volatiles, and hydrolysis of cell wall (Lang et al., 2017 ), important information that can be applied to create a more flavorful tomato fruit. Although research on tomato has been focused on unraveling the influence of epi-mutations on major ripening regulators, the extent and role of epigenetic regulation of ripening, particularly in relation to improving nutrition and flavor, is begging for attention. Likewise, the roles of other genetic players such as histone modification, DNA methylation, chromatin remodeling, and microRNAs in the biosynthesis, regulation, conjugation, transport, and signal transduction of plant hormones in tomato are still poorly understood.
Tomatoes and the Environment
Environmental extremes such as drought, cold, heat, salinity, and other negative conditions impact plant growth, health, and productivity, and this is true for tomato plants as well. Much scientific effort has gone into understanding responses of plants to these stresses (Gerszberg & Hnatuszko-Konka, 2017 ). These approaches include engineering genes encoding functional and structural proteins, genes involved in regulatory and signaling pathways, transcription factors (Wang et al., 2015 ), and stress mitigating enzymes (Lim, Jeong, Jung, & Harn, 2016 ; Lyu et al., 2013 ; Vincour & Altman, 2005 ; Wang, Zhang, Liu, & Li, 2011 ) as well as higher expression of stress hormones and their receptors (Fabregas et al., 2018 ; Upadhyay & Mattoo, 2018 ). Thus a huge body of novel information has accumulated about different players that directly or indirectly impart tolerance to abiotic stresses. These include proteins such as dehydrins, heat shock proteins and late embryogenesis abundant proteins, osmolytes (trehalose and glycine betaine, signaling molecules) polyamines, and hormones (ABA, ethylene and methyl jasmonate) that have been validated (see Mattoo, 2014 , and references therein). Also, genes that respond to stress have been identified by comparative transcriptomics. Those validated for more than a single abiotic stress are transcription factors b-ZIP, ERF/AP2 family, DOF, HD-ZIP, MYB, NAC, WRKY, and Zn-finger, which regulate endogenous processes in plants (reviewed in Shukla & Mattoo, 2013 ; see also Mattoo et al., 2014 ). Successful efforts on engineering tomato plants for resistance to different abiotic stresses have started paying off, as industry has already generated some drought-resistant germplasm for the farmers.
Trichomes, Herbivory, and Defense
To prevent plants from herbivores and mechanical wounding, tomato plants utilize trichomes, which abound epidermally on their leaves and stems (Huchelmann, Boutry, & Hachez, 2017 ; Shepherd & Wagner, 2007 ). Trichomes become a trap as well as a physical hindrance against the herbivory of leaves and stem by small insects (Lobato-Ortiz, Schuster, & Mutschler, 2007 ; Simmons, Gurr, McGrath, Martin, & Nicol, 2004 ). Seven types of trichomes are present in tomato plants, including glandular trichomes (types VI and VII), which comprise four- to eight-celled heads (Thipyapong, Joel, & Steffens, 1997 ). The shape of the head of type VI glandular trichomes differs in wild accessions and modern-day tomato cultivars (Bergau et al., 2015 ). Water-deficient conditions result in higher trichome density in tomato plants compared to well-watered conditions (Galdon-Armero et al., 2018 ). In addition to mitigating water stress conditions, the trichomes create hinderance for insect movement by producing sticky exudates, toxicants, and antinutritional proteins and repellents on aerial surfaces (Figure 6 ) (Frelichowski & Juvik, 2001 ; Lobato-Ortiz et al., 2007 ; Simmons et al., 2004 ; Thipyapong et al., 1997 ).
Figure 6. An illustration of defense mechanisms in tomato against insect or pathogen invasion. Various endogenous compounds (acyl sugars, α -tomatine, SAPs, SLs) are associated with defense response against pathogen infection. NBS-LRR proteins regulate signal transduction pathways leading to pest or disease resistance. MicroRNAs negatively regulate expression of NBS-LRRs whereas lncRNAs modulate effects of miRNAs. Proteinase inhibitors protect plant tissues from insect attack by inhibiting activity of proteases in insect guts. Upon recognition of pathogenic effector (Avr) proteins, plant resistance (R) proteins activate MAPKKK ε which activates a MEK2, SIPK, and WIPK cascade to establish hypersensitive response cell death. The miR164 post-transcriptionally regulates ORE1, which responds to senescence-inducing stimuli and triggers senescence process. Promotive or inhibitory effect is marked with arrows or bold-headed lines, respectively. Illustration is developed based on the information available in previous reports. Abbreviations: Avr, pathogenic effector protein; lncRNAs, long noncoding RNAs; MAPKs, mitogen-activated protein kinases; MEK2, MAP kinase kinase 2; miR, microRNA; NBS-LRRs, nucleotide-binding site leucine-rich repeat proteins; ORE1 , NAC TF ORESARA1; R protein, plant resistance proteins; SAPs, stress-associated proteins; SIPK, salicylic acid-induced protein kinase; SLs, stringolactones; WIPK, wound-inducible protein kinase.
These trichomes also produce volatile monoterpenes α - and β -pinene, limonene, and cis - β -ocimene (Li et al., 2004 ), which attract natural enemies of herbivores (Buttery & Ling, 1993 ; Buttery, Ling, & Light, 1987 ). Also, rupture of foliar glandular trichomes releases hydrogen peroxide on leaf surfaces and induces the synthesis of the plant defense hormone jasmonic acid (Wasternack et al., 2006 ). Jasmonic acid promotes synthesis of trichome-derived terpenes (Chen, Klinkhamer, Escobar-Bravo, & Leiss, 2018 ); a tomato regulatory transcription factor SlMYC1 is essential for type VI glandular trichome development (Flood, 2019 ; Xu et al., 2018 ). Strategies to engineer tomato glandular trichomes are also underway to further study diversity in biosynthesis of specialized plant metabolites (Fan, Miller, Liu, Jones, & Last, 2017 ; Kortbeek et al., 2016 ; Schilmiller, Gilgallon, Ghosh, Jones, & Last, 2016 ).
Drought Stress Tolerance
Mannitol, a sugar alcohol synthesized from fructose, is an important osmolyte in plants. Introduction of its heterologous bacterial ( Escherichia coli ) mt1D gene in tomato plants led to the transgenic tomato being tolerant to abiotic stresses drought, cold, and salinity (Khare et al., 2010 ). A strategy that genetically transformed tomato plants with fused trehalose-6-phosphate synthase and trehalose-6-phosphate phosphatase genes also resulted in transgenic plants that had enhanced drought and salt stress tolerance (Lyu et al., 2013 ). Plants that can maintain the activity of vacuolar proton ATPase (V-ATPase) enzyme also have the ability to survive stress conditions—thus overexpression of an apple VHA-B (subunit of the V-ATPase) in tomato led to the accumulation of another stress fighter called proline, and the corresponding transgenic tomato was found to be drought tolerant (Hu et al., 2012 ). Transgenic tomato plants have been developed with tolerance to drought conditions via overexpression of the heterologous transcription factors Osmyb4 gene, encoding MYB (Vannini et al., 2007 ); DREB1A/CBF3 (Rai, Prakash Rai, Rathaur, Kumar, & Singh, 2013 ); and ZAT12 expressing a C2H2 zinc finger (Rai, Singh, & Shah, 2013 ). Dehydrins and late embryogenesis abundant proteins are other stress-relieving molecules that impart tolerance/adaptation to abiotic stressors. For example, transgenic tomato plants overexpressing the dehydrin tas14 gene were tolerant to long-term drought (and salinity) (Muñoz-Mayor et al., 2012 ), and cold-induced SK3-type (ShDHN) dehydrin (from wild tomato— Solanum habrochaites ) enhanced tolerance to multiple abiotic stresses in tomato (Liu, Yu, et al., 2015 ). Constitutive overexpression of a polyamine synthesis gene, arginine decarboxylase PtADC ( Poncirus trifoliate ), in tomato plants imparted enhanced tolerance against drought stress and dehydration and also led to decreased accumulation of reactive oxygen species (ROS) (Wang et al., 2011 ).
Salt Stress Tolerance
Tomato is also sensitive to excessive salt (Foolad, 2007 ). Genes that impact salinity stress include ion transporters, scavengers of ROS, and osmotic homeostasis enablers (reviewed in Kaur et al., 2017 ). Glycine betaine is a quaternary ammonium compound that helps maintain osmotic potential: Overexpression of betaine aldehyde dehydrogenase gene maintained osmotic potential under salt stress in transgenic tomato (Jia, Zhu, Chang, & Li, 2002 ; Moghaieb et al., 2000 ). Genes regulating ion transporters are important in enhancing salt (and drought) tolerance in tomato. Overexpression of antiporter genes seems to have great potential in developing plants that can withstand salt stress. Recent examples can be found in Garcia-Abellan et al. ( 2014 ), Leidi et al. ( 2010 ), and Yarra et al. ( 2012 ). Other examples include overexpression of glyoxalase genes GlyI (glyoxalase I) and GlyII (glyoxalase II) (Álvarez-Viveros et al., 2013 ); overexpression of fatty acid desaturases, which convert 18:2 linoleic acid to 18:3 linolenic acid (Wang et al., 2014 ); and expression of D-galacturonic acid reductase gene ( GalUR ) (Lim et al., 2016 ). The latter transgenic lines were remarkable since they had an added dose of ascorbic acid and chlorophyll under salt stress.
Cold Stress Tolerance
Tomato suffers from chilling injury at temperatures below 13°C, causing substantial economic losses due to fruit quality deterioration, lesion development, loss of seed viability, and uneven ripening. Chilling temperatures alter physiology, metabolism, and macromolecular processes (reviewed in Miura & Furumoto, 2013 ). Attempts to contain chilling injury symptoms and develop cold resistance in tomato have had some success. Thus, tomato (cv. Moneymaker) transformed with a gene that catalyzed the conversion of choline to glycine betaine improved chilling stress tolerance of the transgenic plants (Park, Jenknic, Pino, Murata, & Chen, 2007 ). Genetically engineered tomato plants overexpressing LetAPX (tomato thylakoidal ascorbate peroxidase gene) were found tolerant to cold stress (Wang, Wisniewski, Meilan, Cui, & Fuchigami, 2006 ), but these changes were accompanied by reduction in chlorophyll, glutathione, and APX activities in comparison to the control plants (Duan, Feng, Wang, Li, & Meng, 2012 ). Polyamines are also called stress-imparting molecules and protect plants against different abiotic stresses (Mattoo, 2014 ; Mattoo, Upadhyay, & Rudrabhatla, 2015 ). Tomato fruit engineered for the ripening-associated accumulation of polyamines, spermidine and spermine, when exposed to 2°C chilling temperature, led to the accumulation of a pathogenesis-related protein (PR1b1), which remained abundant in rewarmed chilled fruit for an extended period compared to the control fruit deficient in these two polyamines (Goyal et al., 2016 ). A positive correlation was found between enhanced PR1b1 protein levels, gene transcripts (of MYC2, MYB1 and CBF1 ), and salicylic acid levels in the high spermidine/spermine transgenic tomato fruit (Goyal et al., 2016 ). It was proposed that polyamine-mediated accumulation of PR1b1 protein in rewarmed chilled tomato could be a preemptive plant defense mechanism related to cold stress-induced disease resistance phenomenon, the function and mechanisms of which are yet to be determined (Moyer, Londo, & Gadoury, 2015 ).
Heat Stress Tolerance and Heat Shock Proteins
A short heat (30º–50°C) treatment of tomato fruit delays fruit softening and increases storage/shelf life of tomatoes (Iwahashi & Hosoda, 2000 ; Mama, Yemer, & Woelore, 2016 ). During this response, more than 22% of pericarp proteins were lost, and a new group of proteins to the tune of 1.1% surfaced. These new proteins were identified as antioxidant enzymes, heat shock proteins, and cell-wall related proteins (Iwahashi & Hosoda, 2000 ). Tomato is known to accumulate heat shock proteins upon heat treatment/exposure. Heat shock protein accumulation seems also correlated with chilling tolerance of tomato (Sabahet, Weiss, & Lurie, 1996 ). Tomato harbors clusters of class-I small heat shock chaperone genes that are transiently regulated by ethylene and involve SlMADS-RIN transcription factor (Shukla et al., 2017 ). Another heat shock protein, tomato HSP21, was found to protect photosynthesis from temperature stress (Neta-Sharir et al., 2005 ). In accord with these discoveries, antisense- LeHSP100 transgenic tomatoes had reduced tolerance to heat stress suggesting that LeHSP100/ClpB contribute to the acquisition of thermotolerance (Yang et al., 2006 ). Tomato transformed with MasHSP24.4 (from wild banana) enabled the transgenic tomato to withstand a high temperature of 45°C (Mahesh et al., 2013 ).
Much new data has been unearthed through studies with transgenic tomatoes and new facets of tomato biology unveiled, which can be further utilized for improving the abiotic stress resistance in future cultivars.
Tomatoes and Disease
Biotic stress due to bacterial, insect, viral, or fungal infection/infestation poses a serious threat to crop yield and farm income. Over 40 bacterial and fungal pathogens are known to infect tomato crops (Khaliluev & Shpakovskii, 2013 ). Initial response of plant tissues to pathogens results in innate active or passive defense mechanism to counteract pathogen attack. Understanding pathogen diversification and evolution pattern has been helpful in the development of disease management strategies. With the start of 21st century , gene cloning and transgenic introduction of pathogen-resistant genes/loci via genetic engineering tools have led to the development of tomatoes resistant to specific pathogens (Bergougnoux, 2014 ; Khaliluev & Shpakovskii, 2013 ). Contemporary efforts are also in play to revolutionize conventional breeding programs with advanced biotechnological interventions. For example, Solanum sisymbriifolium is a wild relative of S. lycopersicum and has the potential to resist various pathogens that infect S. lycopersicum . Successful hybridization of S. lycopersicum with Solanum sisymbriifolium was achieved through in vivo crossing and embryo rescue techniques (Piosik, Ruta-Piosik, Zenkteler, & Zenkteler, 2019 ). Such efforts are further paving the way for breeding programs to introduce resistant genes from wild parents into domesticated genotypes. Similarly, incorporation of resistance ( R ) genes from wild tomato species Solanum pimpinellifolium or Solanum pennellii into cultivated tomato has also conferred resistance against Fusarium oxysporum f. sp. lycopersici (Catanzariti, Lim, & Jones, 2015 , and references therein). Earlier reports have comprehensively elaborated the emerging research involving pathogen-plant interactions, components of pathogen resistance pathway, genes conferring resistance in plants against pathogens, and development of pathogen-resistant genotypes (Alkan & Fortes, 2015 ; Bergougnoux, 2014 ; Cui, Tsuda, & Parker, 2015 ; Fatima et al., 2008 ; Gaur, Verma, & Khurana, 2018 ; Karasov, Chae, Herman, & Bergelson, 2017 ; Mattoo & Handa, 2017 ; Parmar et al., 2017 ). This section focuses only on novel developments made in this aspect during last decade.
Insect Infestation
Insect attack on economically important crops causes severe losses in their potential yield. Insects, while causing damage to crops, also transmit viruses. For example, western flower thrips is a notorious carrier of Tomato Spotted Wilt Virus . Plant defense systems include proteinase inhibitors and secondary metabolites, which protect them from insect attack by inhibiting the activity of proteases in insect guts (Figure 2 ). Tomato plants overexpressing cysteine proteinase inhibitor ( CeCPI ) and a fungal chitinase ( PjCHI-1 ) under pMSPOA promoter had detrimental effect on the growth of root-knot nematodes ( Meloidogyne incognita ) feeding on tomato plants (Chan et al., 2015 ).
Stringolactones are phytohormones derived from carotenoids. They positively regulate defense in tomato against root-knot nematodes (Xu et al., 2019 ). Also, basic helix-loop-helix (bHLH) transcription factor MYC2 negatively regulates nematode defense, possibly by mediating hormonal crosstalk among stringolactones, jasmonic acid, and abscisic acid (Xu et al., 2019 ). A comprehensive analysis of 16 genotypes revealed a high association of acyl sugar contents with plant resistance against two-spotted spider mite and whitefly (Maciel et al., 2018 ). Along similar lines, foliar metabolic profiling of tomato lines resistant or susceptible to feeding damage by western flower thrips revealed α -tomatine and a phenolic compound to be potential defensive compounds (Kim et al., 2019 ). During exploration of transgenic opportunities for pest resistance, constitutive overexpression of BtCry2A in tomatoes was found to develop complete resistance in plants against neonate larval instars of tomato fruit borer, Helicoverpa armigera (Hanur, Reddy, Arya, & Reddy, 2015 ).
Tomato roots constantly cooperate with beneficial microorganisms in the soil. Studies on the interactions between plant roots with beneficial rhizosphere microorganism Trichoderma hazianum showed that salicylic acid, ROS, jasmonic acid, and ethylene collectively limit fungal spread and regulate root growth in a step-wise process. After T. harzianum is recognized by tomato roots, salicylic acid and ROS are produced, which limit fungal infection whereas salicylic acid inhibits jasmonate and ethylene production, thereby controlling root colonization. Thereafter, ethylene and auxin induce root growth by stimulating nutrient transport and modifying root architecture (De Palma et al., 2019 ).
Fungal Pathogens
Fusarium oxysporum is a soil-borne fungal pathogen that colonizes in vascular tissues and causes wilting and death of plants, generally referred to as Fusarium wilt disease. In tomato plants, Fusarium oxysporum f. sp. lycopersici ( Fol ) releases specific effector (Avr) proteins into xylem sap. These disulfide-bonded proteins are recognized by the plant resistance (R) proteins and the plant defense response is activated. In tomato, three R proteins (I, I-2, I-3) are known to recognize their respective Avr proteins (Avr1, Avr2, Avr3) produced by Fusarium oxysporum f. sp. lycopersici (Houterman, Cornelissen, & Rep, 2008 ; Houterman et al., 2009 ; Rep et al., 2004 ) (Figure 6 ). Thus various classes of resistance R genes in complex effector-resistance protein interactions are involved in this resistance phenomenon (Catanzariti et al., 2015 ). Recent developments include the role of micro RNA in defense. Transcripts of two microRNAs, miR482f and miR5300, were found to be associated with susceptibility of tomato cultivars to Fusarium oxysporum f. sp. lycopersici (Ouyang et al., 2014 ). Mimic RNAs (of miR482/2118) enhance tomato resistance against infection with oomycete Phytophthora infestans and bacterial pathogen Pseudomonas syringae pv. Tomato DC3000 (Canto-Pastor et al., 2019 ). Also, tomato plants expressing a fungal gene ( FvC5SD ), which encodes iron-binding protein C-5 sterol desaturase in the ergosterol biosynthesis pathway, led to improved protection against Sclerotinia sclerotiorum due to deposition of their leaves with ~23% more epicuticular wax than control plants (Kamthan et al., 2012 ). These transgenic plants were found more resistant to drought, and fruits had enhanced content of iron and ω -3 and ω -6 polyunsaturated fatty acids (Kamthan et al., 2012 ). Among various exogenous application studies, NO increases tomato plant’s resistance against gray mold rot caused by Botrytis cinerea (Lai et al., 2011 ). Exogenous application of oxalic acid has been shown to induce resistance in tomato plants against Botrytis cinerea by increasing expression of oxalate oxidase and Germin in tomato (Sun et al., 2019 ). Interestingly, exogenous application of chitosan nanoparticles enhances biotic tolerance in tomato against Fusarium andiyazi , involving upregulation of superoxide dismutase gene and pathogenesis-related genes (PR-1, PR-2/ β -1,3-glucanase, PR-8/chitinase, PR-10) (Chun & Chandrasekaran, 2019 ).
Bacterial Pathogens
Pseudomonas syringae pv. tomato causes bacterial spot disease in tomato. Transgenesis has the potential to develop resistance in tomato plants against such bacterial pathogens. Thus over- or misexpression of potato polyphenol oxidase ( StPPO ) in tomato led to reduction in incidence of Pseudomonas syringae pv. tomato infection (Li & Steffens, 2002 ; Thipyapong, Hunt, & Steffens, 2004 ). Similarly, transgenic overexpression of human cathelicidin antimicrobial peptide (hCAP18/LL-37) upregulated expression of pathogenesis-related protein (AT4G25780), bifunctional inhibitor/lipid transfer protein (AT5G38195), and cysteine-rich antifungal protein precursor (AT1G75830), which resulted in 15% growth inhibition of bacterial soft rot, caused by Pectobacterium carotovorum ssp. Carotovorum , and 35% growth inhibition of bacterial spot, caused by Xanthomonas campestris pv. Vesicatoria (Jung, 2013 ). Mitogen-activated protein (MAP) kinase cascade is associated with a signaling pathway related to plant immunity (Figure 6 ). A MAPKKK gene, SlMAPKKK ε , has been identified to induce hypersensitive response cell death by activating a MAP kinase module and establish disease resistance in tomato plants against Xanthomonas campestris and Pseudomonas syringae strains (gram-negative bacterial pathogens) (Melech-Bonfil & Sessa, 2010 ).
Viral Pathogens
An efficient multiplex (RT-PCR) system to detect multiple viral diseases has been established. This system simultaneously detects Tobacco mosaic virus , Cucumber mosaic virus , Tomato Spotted Wilt Virus , Tomato chlorosis virus , Potato virus Y , and Potato virus X in tomato plants (Liu et al., 2019 ). Perturbations in transcript levels of some long noncoding RNAs have also been noticed in tomato plants infected with Tomato yellow leaf curl virus (Zhou et al., 2019 ).
World population is on the rise, as are concerns about production of enough food for the growing population. The educated consumer is concerned about food nutrition, and there is growing demand for health-enhancing fruits and vegetables. Major breakthroughs in sustainable agriculture, gene engineering technologies, and knowledge about nutritional enhancement of produce such as fruits and vegetables are valuable to develop new and valuable germplasm for human health purposes. Tomato is a common vegetable whose fruit has been engineered for higher levels and higher quality of nutrition-rich molecules, including carotenoids (lycopene in particular), vitamins including ascorbic acid, flavonoids, and polyphenols. Moreover, sustainable agricultural practices have been tested to determine the effect of varying agroecosystems on the quality of nonengineered and engineered tomato germplasm. Tomato is one of the major horticultural crops in regard to its consumption and its production volume in the world, cultivated on an area of ~4.8 million hectares with an annual production of 182 million tons. In addition to efforts to find mechanisms and processes to increase fruit yield and longer shelf life, the consumer’s recognition of fruits, including tomato fruit, as sources of health-promoting nutrients has intensified research on tomato. Excellent published research has unraveled dynamic physiological and biochemical changes during ripening of tomato while major advances have been made in the identification of gene regulators and hormonal crosstalk. The valuable information of players including transcription factors, microRNAs, signaling molecules, and their crosstalk at genetic and epigenetic levels is also being utilized toward understanding responses of tomato to vagaries of nature such as stresses due to cold and hot temperatures (both decrease the yield of tomatoes), drought, salinity, and herbivory. Thus genetic and biochemical determinants of pathways impacted by specific abiotic and biotic stressors are being unraveled that should help in developing new and engineered tomato plants that can withstand these stresses.
Acknowledgments
This article was prepared in the absence of any financial or commercial relationships that could be constructed as a potential conflict of interest. All authors equally contributed to writing, editing, commenting, revising and approving the manuscript. We acknowledge research work of all colleagues who have contributed to the understanding of fruit ripening and plant senescence. Due to a vast amount of literature in the subject matter, the authors apologize to those authors whose work was not included in this article. Autar K. Mattoo’s research is supported by United States Department of Agriculture.
Digital Materials
- World Food Preservation Center .
Further Reading
- Mattoo, A. K. , & Handa, A. K. (Eds.). (2017). Achieving sustainable cultivation of tomatoes . Cambridge, U.K.: Burleigh Dodds Science Publishing.
- Nath, N. , Bouzayen, M. , Mattoo, A. K. , & Pech, J.-C. (Eds.). (2014). Fruit ripening: Physiology, signalling and genomics . Oxford, U.K.: CABI.
- Paliyath, G. , Subramanian, J. , Lim, L.-T. , Subramanian, K. S. , Handa, A. K. , & Mattoo, A. K. (Eds.). (2019). Postharvest biology and nanotechnology . New York, NY: John Wiley.
- Aboul-Soud, M. A. M. (2010). Exogenous nitric oxide has negative impacts on ethylene emissions from intact and fresh-cut tomato fruit. Journal of Horticultural Science & Biotechnology , 85 , 516–520.
- Agarwal, S. , & Rao, A. V. (2000). Tomato lycopene and its role in human health and chronic diseases. Canadian Medical Association Journal , 163 , 739–744.
- Alćazar, R. , Altabella, T. , Marco, F. , Bortolotti, C. , Reymond, M. , Koncz, C. , . . . Tiburcio, A. F. (2010). Polyamines: Molecules with regulatory functions in plant abiotic stress tolerance. Planta , 231 , 1237–1249.
- Alkan, N. , & Fortes, A. M. (2015). Insights into molecular and metabolic events associated with fruit response to post-harvest fungal pathogens . Frontiers in Plant Science , 6 , 889.
- Alvarez Viveros, M. F. , Inostroza-Blancheteau, C. , Timmermann, T. , González, M. , & Arce-Johnson, P. (2013). Overexpression of GlyI and GlyII genes in transgenic tomato ( Solanum lycopersicum Mill.) plants confers salt tolerance by decreasing oxidative stress . Molecular Biology Reports , 40 , 3281–3290.
- Anwar, R. , Mattoo, A. K. , & Handa, A. K. (2015). Polyamine interactions with plant hormones: Crosstalk at several levels. In T. Kusano & H. Suzuki (Eds.), Polyamines: A universal molecular nexus for growth, survival, and specialized metabolism (pp. 267–302). London, U.K.: Springer.
- Anwar, R. , Mattoo, A. K. , & Handa, A. K. (2019). Ripening and senescence of fleshy fruits. In G. Paliyath , J. Subramanian , L.-T. Lim , K. S. Subramanian , A. K. Handa , & A. K. Mattoo (Eds.), Postharvest biology and nanotechnology (pp. 15–51). Hoboken, NJ: John Wiley.
- Audran-Delalande, C. , Bassa, C. , Mila, I. , Regad, F. , Zouine, M. , & Bouzayen, M. (2012). Genome-wide identification, functional analysis and expression profiling of the Aux/IAA gene family in tomato. Plant and Cell Physiology , 53 , 659–672.
- Bai, J. , Baldwin, E. A. , Imahori, Y. , Kostenyuk, I. , Burns, J. , & Brecht, J. K. (2011). Chilling and heating may regulate C6 volatile aroma production by different mechanisms in tomato ( Solanum lycopersicum ) fruit . Postharvest Biology and Technology , 60 , 111–120.
- Bailey, L. H. (1896). The philosophy of species-making. Botanical Gazette , 22 , 454–462.
- Baldwin, I. T. , Kessler, A. , & Halitschke, R. (2002). Volatile signaling in plant-plant-herbivore interactions: What is real? Current Opinion in Plant Biology , 5 , 351–354.
- Bapat, V. A. , Trivedi, P. K. , Ghosh, A. , Sane, V. A. , Ganapathi, T. R. , & Nath, P. (2010). Ripening of fleshy fruit: Molecular insight and the role of ethylene . Biotechnology Advances , 28 , 94–107.
- Bergau, N. , Bennewitz, S. , Syrowatka F. , Hause G. , & Tissier, A. (2015). The development of type VI glandular trichomes in the cultivated tomato Solanum lycopersicum and a related wild species S. Habrochaites . BMC Plant Biology , 15 , 289.
- Bergougnoux, V. (2014). The history of tomato: From domestication to biopharming. Biotechnology Advances , 32 , 170–189.
- Bodanapu, R. , Gupta, S. K. , Basha, P. O. , Sakthivel, K. , Sadhana , Sreelakshmi, Y. , & Sharma, R. (2016). Nitric oxide overproduction in tomato shr mutant shifts metabolic profiles and suppresses fruit growth and ripening. Frontiers in Plant Science , 7 , 1714.
- Bouzayen, M. , Latche, A. , Nath, P. , & Pech, J. C. (2010). Mechanism of fruit ripening. Plant Developmental Biology—Biotechnological Perspectives , 1 , 319–339.
- Bovy, A. , de Vos, R. , Kemper, M. , Schijlen, E. , Almenar Pertejo, M. , Muir, S. , . . . van Tunen, A. (2002). High-flavonol tomatoes resulting from the heterologous expression of the maize transcription factor genes LC and C1 . The Plant Cell , 14 (10), 2509–2526.
- Brooks, C. , Nekrasov, V. , Lippman, Z. B. , & Van Eck, J. (2014). Efficient gene editing in tomato in the first generation using the clustered regularly interspaced short palindromic repeats/CRISPR-associated9 system. Plant Physiology , 166 , 1292–1297.
- Bulley, S. , & Laing, W. (2016). The regulation of ascorbate biosynthesis. Current Opinion in Plant Biology , 33 , 15–22.
- Burns, J. , Fraser, P. D. , & Bramley, P. M. (2003). Identification and quantification of carotenoids, tocopherols and chlorophylls in commonly consumed fruits and vegetables. Phytochemistry , 62 , 939–947.
- Butelli, E. , Titta, L. , Giorgio, M. , Mock, H.-P. , Matros, A. , Peterek, S. , . . . Martin, C. (2008). Enrichment of tomato fruit with health-promoting anthocyanins by expression of select transcription factors. Nature Biotechnology , 26 , 1301–1308.
- Buttery, R. G. , & Ling, L. C. (1993). Volatile components of tomato fruit and plant-parts—relationship and biogenesis. ACS Symposium Series , 525 , 23–34.
- Buttery, R. G. , Ling, L. C. , & Light, D. M. (1987). Tomato leaf volatile aroma components. Journal of Agricultural and Food Chemistry , 35 , 1039–1042.
- Canto-Pastor, A. , Santos, B. A. M. C. , Valli, A. A. , Summers, W. , Schornack, S. , & Baulcombe, D. C. (2019). Enhanced resistance to bacterial and oomycete pathogens by short tandem target mimic RNAs in tomato. Proceedings of the National Academy of Sciences , 116 (7), 2755–2760.
- Casals, J. , Rivera, A. , Sabaté, J. , Romero del Castillo, R. , & Simó, J. (2018). Cherry and fresh market tomatoes: Differences in chemical, morphological, and sensory traits and their implications for consumer acceptance. Agronomy , 9 (1), 9.
- Catanzariti, A.-M. , Lim, G. T. T. , & Jones, D. A. (2015). The tomato I-3 gene: A novel gene for resistance to Fusarium wilt disease. New Phytologist , 207 , 106–118.
- Causse, M. , Giovannoni, J. , Bouzayen, M. , & Zouine, M. (Eds.). (2016). The tomato genome . Berlin, Germany: Springer.
- Chan, Y.-L. , He, Y. , Hsiao, T.-T. , Wang, C.-J. , Tian, Z. , & Yeh, K.-W. (2015). Pyramiding taro cystatin and fungal chitinase genes driven by a synthetic promoter enhances resistance in tomato to root-knot nematode Meloidogyne incognita . Plant Science , 231 , 74–81.
- Chen, G. , Klinkhamer, P. G. L. , Escobar-Bravo, R. , & Leiss, K. A. (2018). Type VI glandular trichome density and their derived volatiles are differently induced by jasmonic acid in developing and fully developed tomato leaves: Implications for thrips resistance. Plant Science , 276 , 87–98.
- Chen, G. P. , Hackett, R. , Walker, D. , Taylor, A. , Lin, Z. F. , & Grierson, D. (2004). Identification of a specific isoform of tomato lipoxygenase ( TomloxC ) involved in the generation of fatty acid-derived flavor compounds. Plant Physiology , 136 , 2641–2651.
- Chen, W. , Kong, J. , Qin, C. , Yu, S. , Tan, J. , Chen, Y.-R. , . . . Hong, Y. (2015). Requirement of CHROMOMETHYLASE3 for somatic inheritance of the spontaneous tomato epimutation Colourless non-ripening . Scientific Reports , 5 , 9192.
- Cherian, S. , Figueroa, C. R. , & Nair, H. (2014). “Movers and shakers” in the regulation of fruit ripening: A cross-dissection of climacteric versus non-climacteric fruit. Journal of Experimental Botany , 65 , 4705–4722.
- Chun, S.-C. , & Chandrasekaran, M. (2019). Chitosan and chitosan nanoparticles induced expression of pathogenesis-related proteins genes enhances biotic stress tolerance in tomato. International Journal of Biological Macromolecules , 125 , 948–954.
- Cin, V. D. , Tieman, D. M. , Tohge, T. , McQuinn, R. , de Vos, R. C. H. , Osorio, S. , . . . Klee, H. J. (2011). Identification of genes in the phenylalanine metabolic pathway by ectopic expression of a MYB transcription factor in tomato fruit. The Plant Cell , 23 , 2738–2753.
- Cruz, A. B. , Bianchetti, R. E. , Alves, F. R. R. , Purgatto, E. , Peres, L. E. P. , Rossi, M. , . . . Freschi, L. (2018). Light, ethylene and auxin signaling interaction regulates carotenoid biosynthesis during tomato fruit ripening. Frontiers in Plant Science , 9 , 1370.
- Cui, H. , Tsuda, K. , & Parker, J. E. (2015). Effector-triggered immunity: From pathogen perception to robust defense. Annual Review of Plant Biology , 66 , 487–511.
- Dai, Z. , Wu, H. , Baldazzi, V. , van Leeuwen, C. , Bertin, N. , Gautier, H. , . . . Génard, M. (2016). Inter-species comparative analysis of components of soluble sugar concentration in fleshy fruits. Frontiers in Plant Science , 7 , 649.
- D’Ambrosio, C. , Giorio, G. , Marino, I. , Merendino, A. , Petrozza, A. , Salfi, L. , . . . Cellini, F. (2004). Virtually complete conversion of lycopene into β -carotene in fruits of tomato plants transformed with the tomato lycopene β -cyclase ( tlcy-b ) cDNA. Plant Science , 166 , 207–214.
- Dardick, C. , & Callahan, A. (2014). Evolution of the fruit endocarp: Molecular mechanisms underlying adaptations in seed protection and dispersal strategies. Frontiers in Plant Science , 5 , 284.
- De la Garza, R. I. D. , Gregory, J. F. , & Hanson, A. D. (2007). Folate biofortification of tomato fruit . Proceedings of the National Academy of Science, U.S.A. , 10, 4218–4222.
- De Palma, M. , Salzano, M. , Villano, C. , Aversano, R. , Lorito, M. , Ruocco, M. , . . . Tucci, M. (2019). Transcriptome reprogramming, epigenetic modifications and alternative splicing orchestrate the tomato root response to the beneficial fungus Trichoderma harzianum . Horticulture Research , 6 , 5.
- Di Matteo, A. , Sacco, A. , Anacleria, M. , Pezzotti, M. , Delledonne, M. , Ferrarini, A. , . . . Barone, A. (2010). The ascorbic acid content of tomato fruits is associated with the expression of genes involved in pectin degradation. BMC Plant Biology , 10 , 163.
- D’Introno, A. , Paradiso, A. , Scoditti, E. , D’Amico, L. , De Paolis, A. , Carluccio, M. A. , . . . Giovinazzo, G. (2009). Antioxidant and anti-inflammatory properties of tomato fruits synthesizing different amounts of stilbenes. Plant Biotechnology Journal , 7 , 422–429.
- Duan, M. , Feng, H. L. , Wang, L. Y. , Li, D. , & Meng, Q. W. (2012). Overexpression of thylakoidal ascorbate peroxidase shows enhanced resistance to chilling stress in tomato. Journal of Plant Physiology , 169 , 867–877.
- Eum, H. L. , Kim, H. B. , Choi, S. B. , & Lee, S. K. (2009). Regulation of ethylene biosynthesis by nitric oxide in tomato ( Solanum lycopersicum L.) fruit harvested at different ripening stages. European Food Research and Technology , 228 , 331–338.
- Fabregas, N. , Lozano-Elena, F. , Blasco-Escamez, D. , Tohge, T. , Martinez-Andujar, C. , Albacete, A. , . . . Cano-Delgado, A. I. (2018). Overexpression of the vascular brassinosteroid receptor BRL3 confers drought resistance without penalizing plant growth. Nature Communications, 9 , 4680.
- Fan, P. , Miller, A. M. , Liu, X. , Jones, A. D. , & Last, R. L. (2017). Evolution of a flipped pathway creates metabolic innovation in tomato trichomes through BAHD enzyme promiscuity. Nature Communications , 8 , 2080.
- Fantini, E. , Falcone, G. , Frusciante, S. , Giliberto, L. , & Giuliano, G. (2013). Dissection of tomato lycopene biosynthesis through virus-induced gene silencing. Plant Physiology , 163 , 986.
- FAO . (2016). FAOSTAT. In Food and agriculture organization of the United Nations . Rome, Italy.
- FAO . (2017). FAOSTAT. In Food and agriculture organization of the United Nations . Rome, Italy.
- Farinati, S. , Rasori, A. , Varotto, S. , & Bonghi, C. (2017). Rosaceae fruit development, ripening and post-harvest: An epigenetic perspective. Frontiers in Plant Science , 8 , 1247.
- Fatima, T. , Mattoo, A. K. , Rivera-Domínguez, M. , Troncoso-Rojas, R. , Tiznado-Hernández, M.-E. , & Handa, A. K. (2008). Tomato. In C. Kole & T. C. Hall (Eds.), Compendium of transgenic crop plants (pp. 1–46). Oxford, U.K.: Wiley-Blackwell.
- Fatima, T. , Sobolev, A. P. , Teasdale, J. R. , Kramer, M. , Bunce, J. , Handa, A. K. , . . . Mattoo, A. K. (2016). Fruit metabolite networks in engineered and non-engineered tomato genotypes reveal fluidity in a hormone and agroecosystem specific manner. Metabolomics , 12 , 103.
- Flood, P. J. (2019). The smell of transcription: The SlMYC1 transcription factor makes tomato plants smelly. The Plant Cell , 31 , 2.
- Foolad, M. (2007). Current status of breeding tomatoes for salt and drought tolerance. In M. A. Jenks , P. Hasegawa , & S. M. Jain (Eds.), Advances in molecular breeding toward drought and salt tolerant crops (pp. 669–700). The Netherlands: Springer.
- Fraser, P. D. , Romer, S. , Shipton, C. A. , Mills, P. B. , Kiano, J. W. , Misawa, N. , . . . Bramley, P. M. (2002). Evaluation of transgenic tomato plants expressing an additional phytoene synthase in a fruit-specific manner . Proceedings of the National Academy of Sciences , 99 (2), 1092–1097.
- Frelichowski, J. E. , & Juvik, J. A. (2001). Sesquiterpene carboxylic acids from a wild tomato species affect larval feeding behavior and survival of Helicoverpa zea and Spodoptera exigua (Lepidoptera: Noctuidae). Journal of Economic Entomology , 94 , 1249–1259.
- Friedman, M. (2013). Anticarcinogenic, cardioprotective, and other health benefits of tomato compounds lycopene, α -tomatine, and tomatidine in pure form and in fresh and processed tomatoes. Journal of Agricultural and Food Chemistry , 61 , 9534–9550.
- Fujisawa, M. , Nakano, T. , Shima, Y. , & Ito, Y. (2013). A large-scale identification of direct targets of the tomato MADS Box transcription factor RIPENING INHIBITOR reveals the regulation of fruit ripening. The Plant Cell , 25 , 371–386.
- Galdon-Armero, J. , Fullana-Pericas, M. , Mulet, P. A. , Conesa, M. A. , Martin, C. , & Galmes, J. (2018). The ratio of trichomes to stomata is associated with water use efficiency in Solanum lycopersicum (tomato). The Plant Journal , 96 , 607–619.
- Gallusci, P. , Hodgman, C. , Teyssier, E. , & Seymour, G. B. (2016). DNA methylation and chromatin regulation during fleshy fruit development and ripening. Frontiers in Plant Science , 7 , 807.
- Galpaz, N. , Wang, Q. , Menda, N. , Zamir, D. , & Hirschberg, J. (2008). Abscisic acid deficiency in the tomato mutant high-pigment 3 leading to increased plastid number and higher fruit lycopene content. The Plant Journal , 53 , 717–730.
- Garcia, V. , Stevens, R. , Gil, L. , Gilbert, L. , Gest, N. , Petit, J. , . . . Rothan, C. (2009). An integrative genomics approach for deciphering the complex interactions between ascorbate metabolism and fruit growth and composition in tomato. Comptes Rendus Biologies , 332 , 1007–1021.
- Garcia-Abellan, J. O. , Egea, I. , Pineda, B. , Sanchez-Bela, P. , Belver, A. , Garcia-Sogo, B. , . . . Bolarin, M. C. (2014). Heterologous expression of the yeast HAL5 gene in tomato enhances salt tolerance by reducing shoot Na + accumulation in the long term . Physiologia Plantarum , 152 , 700–713.
- García-Mier, L. , Guevara-González, R. G. , Mondragón-Olguín, V. M. , Del Rocío Verduzco-Cuellar, B. , & Torres-Pacheco, I. (2013). Agriculture and bioactives: Achieving both crop yield and phytochemicals . International Journal of Molecular Sciences , 14 , 4203.
- Garchery, C. , Gest, N. , Do, P. T. , Alhagdow, M. , Baldet, P. , Menard, G. , . . . Stevens, R. (2013). A diminution in ascorbate oxidase activity affects carbon allocation and improves yield in tomato under water deficit. Plant Cell & Environment , 36 , 159–175.
- Gaur, R. K. , Verma, R. K. , & Khurana, S. M. P. (2018). Genetic engineering of horticultural crops: Present and future. In G. R. Rout & K.V. Peter (Eds.), Genetic engineering of horticultural crops (pp. 23–46). New York, NY: Academic Press.
- Gerster, H. (1997). The potential role of lycopene for human health. Journal of the American College of Nutrition , 16 , 109–126.
- Gerszberg, A. , & Hnatuszko-Konka, K. (2017). Tomato tolerance to abiotic stress: A review of most often engineered target sequences. Plant Growth Regulation, 83 , 175.
- Giovannoni, J. , Nguyen, C. , Ampofo, B. , Zhong, S. , & Fei, Z. (2017). The epigenome and transcriptional dynamics of fruit ripening. Annual Review of Plant Biology , 68 , 61–84.
- Giovannucci, E. (1999). Tomatoes, tomato-based products, lycopene, and cancer: Review of the epidemiologic literature. Journal of the National Cancer Institute , 91 , 317–331.
- Giovannucci, E. , Rimm, E. B. , Liu, Y. , Stampfer, M. J. , & Willett, W. C. (2002). A prospective study of tomato products, lycopene, and prostate cancer risk. Journal of the National Cancer Institute , 94 (5), 391–398.
- Giovinazzo, G. , D’Amico, L. , Paradiso, A. , Bollini, R. , Sparvoli, F. , & DeGara, L. (2005). Antioxidant metabolite profiles in tomato fruit constitutively expressing the grapevine stilbene synthase gene. Plant Biotechnology Journal , 3 , 57–69.
- Goyal, R. K. , Fatima, T. , Topuz, M. , Bernadec, A. , Sicher, R. , Handa, A. K. , & Mattoo, A. K. (2016). Pathogenesis-related protein 1b1 (PR1b1) is a major tomato fruit protein responsive to chilling temperature and upregulated in high polyamine transgenic genotypes . Frontiers in Plant Science , 7 , 901.
- Guo, F. , Zhou, W. J. , Zhang, J. C. , Xu, Q. , & Deng, X. X. (2012). Effect of the citrus lycopene β -cyclase transgene on carotenoid metabolism in transgenic tomato fruits. PLoS One , 7 , e32221.
- Handa, A. , Nambeesan, S. , Mengiste, T. , Laluk, K. , AbuQamar, S. , & Mattoo, A. (2010). Polyamine spermidine is an upstream negator of ethylene-regulated pathogenesis of Botrytis cinerea in tomato leaf. Acta Horticulturae , 914 , 109–112.
- Handa, A. K. , Anwar, R. , & Mattoo, A. K. (2014). Biotechnology of fruit quality. In P. Nath , M. Bouzayen , A. K. Mattoo , & J. C. Pech (Eds.), Fruit ripening: Physiology, signalling and genomics (pp. 259–290). Oxford, U.K.: CABI.
- Handa, A. K. , Srivastava, A. , Deng, Z. , Gaffe, J. , Arora, A. , Hernandez, M. E. T. , . . . Mattoo, A. K. (2010). Biotechnological interventions to improve plant developmental traits. In C. Kole , C. H. Michler , A. G. Abbott , & T. C. Hall (Eds.), Transgenic crop plants: Utilization and biosafety (Vol. 2, pp. 199–248). Heidelberg, Germany: Springer-Verlag.
- Handa, A. K. , Tiznado-Hernández, M.-E. , & Mattoo, A. K. (2012). Fruit development and ripening: A molecular perspective. In A. Altman & P. M. Hasegawa (Eds.), Plant biotechnology and agriculture: Prospects for the 21st century (pp. 405–424). New York, NY: Elsevier.
- Hanur, V. , Reddy, B. , Arya, V. , & Reddy, P. V. (2015). Genetic transformation of tomato using Bt Cry2A gene and characterization in Indian cultivar Arka Vikas. Journal of Science and Technology of Agriculture and Natural Resources , 17 , 1805–1814.
- Harpaz-Saad, S. , Yoon, G. M. , Mattoo, A. K. , & Kieber, J. J. (2012). The Formation of ACC and competition between polyamines and ethylene for SAM. Annual Plant Reviews , 44 , 53–81.
- Hiwasa-Tanase, K. , & Ezura, H. (2014). Climacteric and non-climacteric ripening. In P. Nath , M, Bouzayen , A. K. Mattoo , & J. C. Pech (Eds.), Fruit ripening: Physiology, signalling and genomics (pp. 1–14). Oxfordshire, U.K.: CABI.
- Houterman, P. M. , Cornelissen, B. J. C. , & Rep, M. (2008). Suppression of plant resistance gene-based immunity by a fungal effector. PLoS Pathogens , 4 , e1000061.
- Houterman, P. M. , Ma, L. , van Ooijen, G. , de Vroomen, M. J. , Cornelissen, B. J. C. , Takken, F. L. W. , & Rep, M. (2009). The effector protein Avr2 of the xylem-colonizing fungus Fusarium oxysporum activates the tomato resistance protein I-2 intracellularly. Plant Journal , 58 , 970–978.
- Huang, X. , Tang, L. , Yu, Y. , Dalrymple, J. , Lippman, Z. B. , & Xu, C. (2018). Control of flowering and inflorescence architecture in tomato by synergistic interactions between ALOG transcription factors. Journal of Genetics and Genomics , 45 , 557–560.
- Huchelmann, A. , Boutry, M. , & Hachez, C. (2017). Plant glandular trichomes: Natural cell factories of high biotechnological interest. Plant Physiology , 175 , 6–22.
- Hu, D. G. , Wang, S. H. , Luo, H. , Ma, Q. J. , Yao, Y. X. , You, C. X. , . . . Hao, Y. J. (2012). Overexpression of MdVHA-B , a V-ATPase gene from apple, confers tolerance to drought in transgenic tomato. Scientia Horticulturae , 145 , 94–101.
- Ioannidi, E. , Kalamaki, M. S. , Engineer, C. , Pateraki, I. , Alexandrou, D. , Mellidou, I. , Giovannonni, J. , & Kanellis, A. K. (2009). Expression profiling of ascorbic acid-related genes during tomato fruit development and ripening and in response to stress conditions. Journal of Experimental Botany , 60 , 663–678.
- Iwahashi, Y. , & Hosoda, H. (2000). Effect of heat stress on tomato fruit protein expression. Electrophoresis , 21 , 1766–1771.
- Jia, G. X. , Zhu, Z. Q. , Chang, F. Q. , & Li, Y. X. (2002). Transformation of tomato with the BADH gene from Atriplex improves salt tolerance . Plant Cell Reports , 21 , 141–146.
- Jones, B. , Frasse, P. , Olmos, E. , Zegzouti, H. , Li, Z. G. , Latché, A. , . . . Bouzayen, M. (2002). Down-regulation of DR12, an auxin-response-factor homolog, in the tomato results in a pleiotropic phenotype including dark green and blotchy ripening fruit. The Plant Journal , 32 , 603–613.
- Jung, Y. J. (2013). Enhanced resistance to bacterial pathogen in transgenic tomato plants expressing cathelicidin antimicrobial peptide. Biotechnology and Bioprocess Engineering , 18 , 615–624.
- Kamthan, A. , Kamthan, M. , Azam, M. , Chakraborty, N. , Chakraborty, S. , & Datta, A. (2012). Expression of a fungal sterol desaturase improves tomato drought tolerance, pathogen resistance and nutritional quality. Scientific Reports , 2 , 951.
- Kanellis, A. K. , & Manganaris, G. A. (2014). Antioxidants and bioactive compounds in fruits. In P. Nath , M. Bouzayen , A. K. Mattoo , & J. C. Pech (Eds.), Fruit ripening: Physiology, signalling and genomics (pp. 99–126). Wallingford, U.K.: CABI.
- Karasov, T. L. , Chae, E. , Herman, J. J. , & Bergelson, J. (2017). Mechanisms to mitigate the trade-off between growth and defense. The Plant Cell , 29 , 666–680.
- Kaur, B. , Handa, A. K. , & Mattoo, A. K. (2017). Genetic engineering of tomato to improve nutritional quality, resilience to abiotic and biotic stresss, and for non-food applications. In A. K. Mattoo & A. K. Handa (Eds.), Achieving sustainable cultivation of tomatoes (pp. 239–281). Cambridge, U.K.: Burleigh Dodds Science Publishing.
- Khaliluev, M. R. , & Shpakovskii, G. V. (2013). Genetic engineering strategies for enhancing tomato resistance to fungal and bacterial pathogens. Russian Journal of Plant Physiology , 60 , 721–732.
- Khare, N. , Goyary, D. , Kumar Singh, N. , Shah, P. , Rathore, M. , Anandhan, S. , . . . Ahmed, Z. (2010). Transgenic tomato cv. Pusa Uphar expressing a bacterial mannitol-1-phosphate dehy- drogenase gene confers abiotic stress tolerance. Plant Cell, Tissue and Organ Culture , 103 , 267–277.
- Kim, H. K. , Vrieling, K. , Klinkhamer, P. G. L. , Mol, S. , Leiss, K. A. , Bac-Molenaar, J. A. , . . . Verlaan, M. G. (2019). Trichome independent resistance against western flower thrips in tomato. Plant & Cell Physiology , 60 , 1011–1024.
- Klee, H. J. , & Giovannoni, J. J. (2011). Genetics and control of tomato fruit ripening and quality attributes. Annual Review of Genetics , 45 , 41–59.
- Klie, S. , Osorio, S. , Tohge, T. , Drincovich, M. F. , Fait, A. , Giovannoni, J. J. , . . . Nikoloski, Z. (2014). Conserved changes in the dynamics of metabolic processes during fruit development and ripening across species. Plant Physiology , 164 , 55–68.
- Kolotilin, I. , Koltai, H. , Bar-Or, C. , Chen, L. , Nahon, S. , Shlomo, H. , . . . Reuveni, M. (2011). Expressing yeast SAMdc gene confers broad changes in gene expression and alters fatty acid composition in tomato fruit. Physiologia Plantarum , 142 , 211–223.
- Kortbeek, R. W. J. , Xu, J. , Ramirez, A. , Spyropoulou, E. , Diergaarde, P. , Otten-Bruggeman, I. , . . . Bleeker, P. M. (2016). Engineering of tomato glandular trichomes for the production of specialized metabolites. In S. E. O’Connor (Ed.), Methods in enzymology (Vol. 576, pp. 305–331). New York, NY: Academic Press.
- Kumar, R. , Khurana, A. , & Sharma, A. K. (2014). Role of plant hormones and their interplay in development and ripening of fleshy fruits. Journal of Experimental Botany , 65 , 4561–4575.
- Lai, T. F. , Wang, Y. Y. , Li, B. Q. , Qin, G. Z. , & Tian, S. P. (2011). Defense responses of tomato fruit to exogenous nitric oxide during postharvest storage. Postharvest Biology and Technology , 62 , 127–132.
- Lander, E. S. (2016). The heroes of CRISPR . Cell , 164 , 18–28.
- Lang, Z. , Wang, Y. , Tang, K. , Tang, D. , Datsenka, T. , Cheng, J. , . . . Zhu, J.-K. (2017). Critical roles of DNA demethylation in the activation of ripening-induced genes and inhibition of ripening-repressed genes in tomato fruit. Proceedings of the National Academy of Sciences , 114 , E4511–E4519.
- Law, J. A. , & Jacobsen, S. E. (2010). Establishing, maintaining and modifying DNA methylation patterns in plants and animals. Nature Reviews Genetics , 11 , 204–220.
- Leidi, E. O. , Barragan, V. , Rubio, L. , El-Hamdaoui, A. , Ruiz, T. M. , Cubero, B. , . . . Pardo, J. M. (2010). The AtNHX1 exchanger mediates potassium compartmentation in vacuoles of transgenic tomato. Plant Journal , 61 , 495–506.
- Leng, P. , Yuan, B. , & Guo, Y. (2014). The role of abscisic acid in fruit ripening and responses to abiotic stress. Journal of Experimental Botany , 65 , 4577–4588.
- Li, L. , & Steffens, J. C. (2002). Overexpression of polyphenol oxidase in transgenic tomato plants results in enhanced bacterial disease resistance. Planta , 215 , 239–247.
- Li, L. , Zhao, Y. , McCaig, B. C. , Wingerd, B. A. , Wang, J. , Whalon, M. E. , . . . Howe, G. A. (2004). The tomato homolog of CORONATINE-INSENSITIVE1 is required for the maternal control of seed maturation, jasmonate-signaled defense responses, and glandular trichome development. The Plant Cell , 16 , 126–143.
- Lim, M. Y. , Jeong, B. R. , Jung, M. , & Harn, C. H. (2016). Transgenic tomato plants expressing strawberry d-galacturonic acid reductase gene display enhanced tolerance to abiotic stresses. Plant Biotechnology Reports , 10 , 105–116.
- Liu, H. , Wu, K. , Wu, W. , Mi, W. , Hao, X. , & Wu, Y. (2019). A multiplex reverse transcription PCR assay for simultaneous detection of six main RNA viruses in tomato plants. Journal of Virological Methods , 265 , 53–58.
- Liu, H. , Yu, C. , Li, H. , Ouyang, B. , Wang, T. , Zhang, J. , . . . Ye, Z. (2015). Overexpression of ShDHN , a dehydrin gene from Solanum habrochaites enhances tolerance to multiple abiotic stresses in tomato. Plant Science , 231 , 198–211.
- Liu, R. H. (2013). Health-promoting components of fruits and vegetables in the diet. Advances in Nutrition , 4 , 384S–392S.
- Liu, R. , How-Kit, A. , Stammitti, L. , Teyssier, E. , Rolin, D. , Mortain-Bertrand, A. , . . . Gallusci, P. (2015). A DEMETER-like DNA demethylase governs tomato fruit ripening. Proceedings of the National Academy of Sciences , 112 , 10804–10809.
- Liu, S. , Yuan, X. , Wang, Y. , Wang, H. , Wang, J. , Shen, Z. , . . . Song, F. (2019). Tomato stress-associated protein 4 contributes positively to immunity against necrotrophic fungus Botrytis cinerea . Molecular Plant-Microbe Interactions , 32 (5), 566–582.
- Llorente, B. , D’Andrea, L. , & Rodríguez-Concepción, M. (2016). Evolutionary recycling of light signaling components in fleshy fruits: New insights on the role of pigments to monitor ripening. Frontiers in Plant Science , 7 , 263.
- Lobato-Ortiz, R. , Schuster, D. J. , & Mutschler, M. A. (2007). Second generation of tomato acylsugar lines with reduction in S. pennellii DNA content are resistant to silverleaf whitefly and improved for fruit characters. HortScience , 42 , 1015.
- Luo, J. , Butelli, E. , Hill, L. , Parr, A. , Niggeweg, R. , Bailey, P. , . . . Martin, C. (2008). AtMYB12 regulates caffeoyl quinic acid and flavonol synthesis in tomato: Expression in fruit results in very high levels of both types of polyphenol. The Plant Journal , 56 , 316–326.
- Lü, P. , Yu, S. , Zhu, N. , Chen, Y.-R. , Zhou, B. , Pan, Y. , . . . Zhong, S. (2018). Genome encode analyses reveal the basis of convergent evolution of fleshy fruit ripening. Nature Plants , 4 , 784–791.
- Luo, Z. D. , Zhang, J. H. , Li, J. H. , Yang, C. X. , Wang, T. T. , Ouyang, B. , . . . Ye, Z. B. (2013). A STAY-GREEN protein SlSGR1 regulates lycopene and beta-carotene accumulation by interacting directly with SlPSY1 during ripening processes in tomato. New Phytologist , 198 , 442–452.
- Lyu, J. I. , Min, S. R. , Lee, J. H. , Lim, Y. H. , Kim, J. K. , Bae, C. H. , & Liu, J. R. (2013). Overexpression of a trehalose-6-phosphate synthase/phosphatise fusion gene enhances tolerance and photosyn- thesis during drought and salt stress without growth aberrations in tomato. Plant Cell, Tissue and Organ Culture , 112 , 257–262.
- Maciel, G. M. , Peixoto, J. , Rocha, J. P. R. , Almeida, R. S. , Oliveira, C. S. , & Mendonça, T. F. N. (2018). Multivariate techniques in the determination of genetic diversity in pest-resistant mini tomato genotypes. Horticultura Brasileira , 36 , 504–509.
- Mahesh, U. , Mamidala, P. , Rapolu, S. , Aragao, F. J. L. , Souza, M. T. , Rao, P. J. M. , . . . Nanna, R. S. (2013). Constitutive overexpression of small HSP24.4 gene in transgenic tomato conferring tolerance to high-temperature stress. Molecular Breeding , 32 , 687–697.
- Mama, S. , Yemer, J. , & Woelore, W. (2016). Effect of hot water treatments on shelf life of tomato (Lycopersicon esculentum Mill). Journal of Natural Sciences Research , 6 , 69–77.
- Manjunatha, G. , Gupta, K. J. , Lokesh, V. , Mur, L. A. J. , & Neelwarne, B. (2012). Nitric oxide counters ethylene effects on ripening fruits. Plant Signaling & Behavior , 7 , 476–483.
- Manjunatha, G. , Lokesh, V. , & Neelwarne, B. (2010). Nitric oxide in fruit ripening: Trends and opportunities. Biotechnology Advances , 28 , 489–499.
- Mattoo, A. K. (2014). Translational research in agricultural biology—enhancing crop resistivity against environmental stress alongside nutritional quality . Frontiers in Chemistry , 2 , 30.
- Mattoo, A. K. , Fatima, T. , Upadhyay, R. K. , & Handa, A. K. (2014). Polyamines in plants: Biosynthesis from arginine, and metabolic, physiological and stress-response roles. In F. D’Mello (Ed.), Amino acids in higher plants (p. 177–194). Oxford, U.K.: CABI.
- Mattoo, A. K. , & Handa, A. K. (2008). Higher polyamines restore and enhance metabolic memory in ripening fruit. Plant Science , 174 , 386–393.
- Mattoo, A. K. , Minocha, S. C. , Minocha, R. , & Handa, A. K. (2010). Polyamines and cellular metabolism in plants: Transgenic approaches reveal different responses to diamine putrescine versus higher polyamines spermidine and spermine. Amino Acids , 38 , 405–413.
- Mattoo, A. K. , Shukla, V. , Fatima, T. , Handa, A. K. , & Yachha, S. K. (2010). Genetic engineering to enhance crop-based phytonutrients (nutraceuticals) to alleviate diet-related diseases. In M. T. Giardi , G. Rea , B. Berra (Eds.), Bio-farms for nutraceuticals: Functional food and safety control by biosensors (Vol. 698, pp. 122–143). Austin, TX: Landes Bioscience.
- Mattoo, A. K. , Sobolev, A. P. , Neelam, A. , Goyal, R. K. , Handa, A. K. , & Segre, A. L. (2006). NMR spectroscopy-based metabolite profiling of transgenic tomato fruit engineered to accumulate spermidine and spermine reveals enhanced anabolic and nitrogen-carbon interactions. Plant Physiology , 142 , 1759–1770.
- Mattoo, A. K. , Upadhyay, R. K. , & Rudrabhatla, S. (2015). Abiotic stress in crops: Candidate genes, osmolytes, polyamines, and biotechnological intervention. In G. K. Pandey (Ed.), Elucidation of abiotic stress signaling in plants (pp. 415–437). New York, NY: Springer Science+Business Media.
- McAtee, P. , Karim, S. , Schaffer, R. J. , & David, K. (2013). A dynamic interplay between phytohormones is required for fruit development, maturation and ripening. Frontiers in Plant Science , 4 , 79.
- Mehta, R. A. , Cassol, T. , Li, N. , Ali, N. , Handa, A. K. , & Mattoo, A. K. (2002). Engineered polyamine accumulation in tomato enhances phytonutrient content, juice quality, and vine life. Nature Biotechnology , 20 , 613–618.
- Melech-Bonfil, S. , & Sessa, G. (2010). Tomato MAPKKK ε is a positive regulator of cell-death signaling networks associated with plant immunity. The Plant Journal , 64 , 379–391.
- Mellidou, I. , & Kanellis, A. K. (2017). Genetic control of ascorbic acid biosynthesis and recycling in horticultural crops. Frontiers in Chemistry , 5 , 50.
- Mellidou, I. , Keulemans, J. , Kanellis, A. K. , & Davey, M. W. (2012). Regulation of fruit ascorbic acid concentrations during ripening in high and low vitamin C tomato cultivars . BMC Plant Biology , 12 , 239.
- Miura, K. , & Furumoto, T. (2013). Cold signaling and cold response in plants. International Journal of Molecular Science , 14 , 5312–5337.
- Moghaieb, R. E. A. , Tanaka, N. , Saneoka, H. , Hussein, H. A. , Yousef, S. S. , Ewada, M. A. F. , . . . Fujita, K. (2000). Expression of betaine aldehyde dehydrogenase gene in transgenic tomato hairy roots leads to the accumulation of glycine betaine and contributes to the maintenance of the osmotic potential under salt stress. Soil Science and Plant Nutrition , 46 , 873–883.
- Mou, W. , Li, D. , Bu, J. , Jiang, Y. , Khan, Z. U. , Luo, Z. , . . . Ying, T. (2016). Comprehensive analysis of ABA effects on ethylene biosynthesis and signaling during tomato fruit ripening. PLoS One , 11 , e0154072.
- Moyer, M. M. , Londo, J. , & Gadoury, D. M. (2015). Cold stress-induced disease resistance (SIDR): Indirect effects of low temperatures on host-pathogen interactions and disease progress in the grapevine powdery mildew pathosystem. European Journal of Plant Pathology , 144 , 695–705.
- Muir, S. R. , Collins, G. J. , Robinson, S. , Hughes, S. , Bovy, A. , Ric De Vos, C. H. , . . . Verhoeyen, M. E. (2001). Overexpression of petunia chalcone isomerase in tomato results in fruit containing increased levels of flavonols. Nature Biotechnology , 19 , 470–474.
- Muñoz-Mayor, M. F. , Pineda, B. , Garcia-Abellán. J. O. , Antón, T. , Garcia-Sogo, B. , Sanchez-Bel, P. , . . . Bolarin, M. C. (2012). Overexpression of dehydrin tas14 gene improves the osmotic stress imposed by drought and salinity in tomato. Journal of Plant Physiology , 169 , 459–468.
- Nambeesan, S. , Datsenka, T. , Ferruzzi, M. G. , Malladi, A. , Mattoo, A. K. , & Handa, A. K. (2010). Overexpression of yeast spermidine synthase impacts ripening, senescence and decay symptoms in tomato. The Plant Journal , 63 , 836–847.
- Neily, M. H. , Matsukura, C. , Maucourt, M. , Bernillon, S. , Deborde, C. , Moing, A. , . . . Ezura, H. (2011). Enhanced polyamine accumulation alters carotenoid metabolism at the transcriptional level in tomato fruit over-expressing spermidine synthase. Journal of Plant Physiology , 168 (3), 242–252.
- Neta-Sharir, I. , Isaacson, T. , Lurie, S. , & Weiss, D. (2005). Dual role for tomato heat shock protein 21: Protecting photosystem II from oxidative stress and promoting color changes during fruit maturation . The Plant Cell , 17 , 1829–1838.
- Osorio, S. , Alba, R. , Damasceno, C. M. B. , Lopez-Casado, G. , Lohse, M. , Zanor, M. I. , . . . Giovannoni, J. J. , & Fernie, A. R. (2011). Systems biology of tomato fruit development: Combined transcript, protein, and metabolite analysis of tomato transcription factor ( nor , rin ) and ethylene receptor ( Nr ) mutants reveals novel regulatory interactions. Plant Physiology , 157 , 405–425.
- Osorio, S. , Alba, R. , Nikoloski, Z. , Kochevenko, A. , Fernie, A. R. , & Giovannoni, J. J. (2012). Integrative comparative analyses of transcript and metabolite profiles from pepper and tomato ripening and development stages uncovers species-specific patterns of network regulatory behavior. Plant Physiology , 159 , 1713–1729.
- Osorio, S. , & Fernie, A. R. (2013). Biochemistry of fruit ripening. In G. B. Seymour (Ed.), The molecular biology and biochemistry of fruit ripening (pp. 1–19). Chichester, U.K.: Wiley-Blackwell.
- Osorio, S. , Scossa, F. , & Fernie, A. (2013). Molecular regulation of fruit ripening. Frontiers in Plant Science , 4 , 1–8.
- Ouyang, S. , Park, G. , Atamian, H. S. , Han, C. S. , Stajich, J. E. , Kaloshian, I. , & Borkovich, K. A. (2014). MicroRNAs suppress NB domain genes in tomato that confer resistance to Fusarium oxysporum . PLoS Pathogens , 10 , e1004464.
- Park, E. J. , Jenknic, Z. , Pino, M. T. , Murata, N. , & Chen, T. H. H. (2007). Glycinebetaine accumulation is more effective in chloroplasts than in the cytosol for protecting transgenic tomato plants against abiotic stress. Plant, Cell & Environment , 30 , 994–1005.
- Parmar, N. , Singh, K. H. , Sharma, D. , Singh, L. , Kumar, P. , Nanjundan, J. , . . . Thakur, A. K. (2017). Genetic engineering strategies for biotic and abiotic stress tolerance and quality enhancement in horticultural crops: a comprehensive review. 3 Biotech , 7 , 239.
- Paul, V. , Pandey, R. , & Srivastava, G. C. (2012). The fading distinctions between classical patterns of ripening in climacteric and non-climacteric fruit and the ubiquity of ethylene—an overview. Journal of Food Science and Technology , 49 , 1–21.
- Peralta, I. E. , Knapp, S. , & Spooner, D. M. (2006). Nomenclature for wild and cultivated tomatoes. TGC Report , 56 , 1–12.
- Peralta, I. E. , & Spooner, D. M. (2007). History, origin and early cultivation of tomato (Solanaceae). In M. K. Razdan & A. K. Mattoo (Eds.), Genetic improvement of solanaceous crops, Vol. 2: Tomato (pp. 1–14). Enfield, NH: Science Publishers.
- Périlleux, C. , Lobet, G. , & Tocquin, P. (2014). Inflorescence development in tomato: Gene functions within a zigzag model. Frontiers in Plant Science , 5 , 121.
- Piosik, Ł. , Ruta-Piosik, M. , Zenkteler, M. , & Zenkteler, E. (2019). Development of interspecific hybrids between Solanum lycopersicum L. and S. sisymbriifolium Lam. via embryo calli. Euphytica , 215 , 31.
- Rai, A. C. , Singh, M. , & Shah, K. (2013). Engineering drought tolerant tomato plants over expressing BcZAT12 gene encoding a C2H2 zinc finger transcription factor. Phytochemistry , 85 , 44–50.
- Rai, G. K. , Prakash Rai, N. , Rathaur, S. , Kumar, S. , & Singh, M. (2013). Expression of r d29A::AtDREB1A / CBF3 in tomato alleviates drought-induced oxidative stress by regulating key enzymatic and non-enzymatic antioxidants. Plant Physiology and Biochemistry , 69 , 90–100.
- Rep, M. , van der Does, H. C. , Meijer, M. , van Wijk, R. , Houterman, P. M. , Dekker, H. L.,. . . Cornelissen, B. J. C. (2004). A small, cysteine-rich protein secreted by Fusarium oxysporum during colonization of xylem vessels is required for I-3-mediated resistance in tomato. Molecular Microbiology , 53 , 1373–1383.
- Rigano, M. M. , Raiola, A. , Docimo, T. , Ruggieri, V. , Calafiore, R. , Vitaglione, P. , . . . Barone, A. (2016). Metabolic and molecular changes of the phenylpropanoid pathway in tomato ( Solanum lycopersicum ) lines carrying different Solanum pennellii wild chromosomal regions. Frontiers in Plant Science , 7 , 1484.
- Roberts, D. P. , & Mattoo, A. K. (2019). Crop production systems, plant metabolic physiology, nutrients, and defining nutritional food quality. Manuscript under review.
- Robertson, L. R. , & Labate, J. A. (2007). Genetic resources of tomato ( Lycopersicon esculentum Mill) and wild relatives. In M. K. Razdan & A. K. Mattoo (Eds.), Genetic improvement of solanaceous crops, Vol. 2: Tomato (pp. 25–75). Enfield, NH: Science.
- Ronen, G. , Carmel-Goren, L. , Zamir, D. , & Hirschberg, J. (2000). An alternative pathway to β -carotene formation in plant chromoplasts discovered by map-based cloning of Beta and old-gold color mutations in tomato. Proceedings of the National Academy of Sciences , 97 , 11102–11107.
- Rosati, C. , Aquilani, R. , Dharmapuri, S. , Pallara, P. , Marusic, C. , Tavazza, R. , . . . Giuliano, G. (2000). Metabolic engineering of beta-carotene and lycopene content in tomato fruit. The Plant Journal , 24 , 413–420.
- Ross, J. A. , & Kasum, C. M. (2002). Dietary flavonoids: Bioavailability, metabolic effects, and safety. Annual Review of Nutrition , 22 , 19–34.
- Rothan, C. , Diouf, I. , & Causse, M. (2018). Trait discovery and editing in tomato. The Plant Journal , 97 , 73–90.
- Ruan, Y.-L. , Patrick, J. W. , Bouzayen, M. , Osorio, S. , & Fernie, A. R. (2012). Molecular regulation of seed and fruit set. Trends in Plant Science , 17 , 656–665.
- Sabahet, A. , Weiss, D. , & Lurie, S. (1996). The correlation between heat-shock protein accumulation and persistence and chilling tolerance in tomato fruit. Plant Physiology , 110 , 531–537.
- Sagar, M. , Chervin, C. , Mila, I. , Hao, Y. W. , Roustan, J. P. , Benichou, M. , . . . Zouine, M. (2013). SlARF4, an auxin response factor involved in the control of sugar metabolism during tomato fruit development. Plant Physiology , 161 , 1362–1374.
- Schijlen, E. , Ric de Vos, C. H. , Jonker, H. , Van Den Broeck, H. , Molthoff, J. , Van Tunen, A. , . . . Bovy, A. (2006). Pathway engineering for healthy phytochemicals leading to the production of novel flavonoids in tomato fruit. Plant Biotechnology Journal , 4 , 433–444.
- Schilmiller, A. L. , Gilgallon, K. , Ghosh, B. , Jones, A. D. , & Last, R. L. (2016). Acylsugar acylhydrolases: Carboxylesterase-catalyzed hydrolysis of acylsugars in tomato trichomes. Plant Physiology , 170 , 1331–1344.
- Schreiber, G. , Reuveni, M. , Evenor, D. , Oren-Shamir, M. , Ovadia, R. , Sapir-Mir, M. , . . . Levin, I. (2012). ANTHOCYANIN1 from Solanum chilense is more efficient in accumulating anthocyanin metabolites than its Solanum lycopersicum counterpart in association with the ANTHOCYANIN FRUIT phenotype of tomato. TAG Theoretical and Applied Genetics , 124 , 295–307.
- Seymour, G. B. , Chapman, N. H. , Chew, B. L. , & Rose, J. K. C. (2013). Regulation of ripening and opportunities for control in tomato and other fruits. Plant Biotechnology Journal , 11 , 269–278.
- Seymour, G. B. , Østergaard, L. , Chapman, N. H. , Knapp, S. , & Martin, C. (2013). Fruit development and ripening. Annual Review of Plant Biology , 64 , 219–241.
- Seymour, G. B. , Poole, M. , Giovannoni, J. J. , & Tucker, G. A. (Eds.). (2013). The molecular biology and biochemistry of fruit ripening . Chichester, U.K.: Wiley-Blackwell.
- Sharma, M. K. , Kumar, R. , Solanke, A. U. , Sharma, R. , Tyagi, A. K. , & Sharma, A. K. (2010). Identification, phylogeny, and transcript profiling of ERF family genes during development and abiotic stress treatments in tomato. Molecular Genetics and Genomics , 284 , 455–475.
- Shepherd, R. W. , & Wagner, G. J. (2007). Phylloplane proteins: Emerging defenses at the aerial frontline? Trends in Plant Science, 12 , 51–56.
- Shukla, V. , & Mattoo, A. K. (2013). Developing robust crop plants for sustaining growth and yield under adverse climatic changes. In N. Tuteja & S. Gill (Eds.), Climate change and plant abiotic stress tolerance (pp. 27–56). Weinheim, Germany: Wiley-VCH Verlag.
- Shukla, V. , Upadhyay, R. K. , Tucker, M. L. , Giovannoni, J. J. , Rudrabhatla, S. V. , & Mattoo, A. K. (2017). Transient regulation of three clustered tomato class-I small heat-shock chaperone genes by ethylene is mediated by SlMADS-RIN transcription factor. Scientific Reports , 7 , 6474.
- Silva Ferreira, D. , Kevei, Z. , Kurowski, T. , de Noronha Fonseca, M. E. , Mohareb, F. , Boiteux, L. S. , & Thompson, A. J. (2018). BIFURCATE FLOWER TRUSS: A novel locus controlling inflorescence branching in tomato contains a defective MAP kinase gene. Journal of Experimental Botany , 69 , 2581–2593.
- Simmons, A. T. , Gurr, G. M. , McGrath, D. , Martin, P. M. , & Nicol, H. I. (2004). Entrapment of Helicoverpa armigera (Hübner) (Lepidoptera: Noctuidae) on glandular trichomes of Lycopersicon species. Australian Journal of Entomology , 43 , 196–200.
- Sobolev, A. P. , Neelam, A. , Fatima, T. , Shukla, V. , Handa, A. K. , & Mattoo, A. K. (2014). Genetic introgression of ethylene-suppressed transgenic tomatoes with higher-polyamines trait overcomes many unintended effects due to reduced ethylene on the primary metabolome. Frontiers in Plant Science , 5 , 632.
- Soyk, S. , Müller, N. A. , Park, S. J. , Schmalenbach, I. , Jiang, K. , Hayama, R. , . . . Lippman, Z. B. (2016). Variation in the flowering gene SELF PRUNING 5G promotes day-neutrality and early yield in tomato. Nature Genetics , 49 , 162.
- Srivastava, A. , Chung, S. H. , Fatima, T. , Datsenka, T. , Handa, A. K. , & Mattoo, A. K. (2007). Polyamines as anabolic growth regulators revealed by transcriptome analysis and metabolite profiles of tomato fruits engineered to accumulate spermidine and spermine. Plant Biotechnology , 24 , 57–70.
- Su, L. , Diretto, G. , Purgatto, E. , Danoun, S. , Zouine, M. , Li, Z. , . . . Chervin, C. (2015). Carotenoid accumulation during tomato fruit ripening is modulated by the auxin-ethylene balance. BMC Plant Biology , 15 , 114.
- Sun, G. , Feng, C. , Zhang, A. , Zhang, Y. , Chang, D. , Wang, Y. , & Ma, Q. (2019). The dual role of oxalic acid on the resistance of tomato against Botrytis cinerea . World Journal of Microbiology and Biotechnology , 35 , 36.
- Sun, L. , Yuan, B. , Zhang, M. , Wang, L. , Cui, M. , Wang, Q. , & Leng, P. (2012). Fruit-specific RNAi-mediated suppression of SlNCED1 increases both lycopene and β -carotene contents in tomato fruit. Journal of Experimental Botany , 63 , 3097–3108.
- Teyssier, E. , Bernacchia, G. , Maury, S. , Kit, A. H. , Stammitti-Bert, L. , Rolin, D. , & Gallusci, P. (2008). Tissue dependent variations of DNA methylation and endoreduplication levels during tomato fruit development and ripening. Planta , 228 , 391–399.
- Thipyapong, P. , Hunt, M. D. , & Steffens, J. C. (2004). Antisense downregulation of polyphenol oxidase results in enhanced disease susceptibility. Planta , 220 , 105–117.
- Thipyapong, P. , Joel, D. M. , & Steffens, J. C. (1997). Differential expression and turnover of the tomato polyphenol oxidase gene family during vegetative and reproductive development. Plant Physiology , 113 , 707–718.
- Tieman, D. , Taylor, M. , Schauer, N. , Fernie, A. R. , Hanson, A. D. , & Klee, H. J. (2006). Tomato aromatic amino acid decarboxylases participate in synthesis of the flavor volatiles 2-phenylethanol and 2-phenylacetaldehyde. Proceedings of the National Academy of Sciences , 103 , 8287–8292.
- Tieman, D. , Zhu, G. , Resende, M. F. R., Jr. , Lin, T. , Nguyen, C. , Bies, D. , . . . Klee, H. (2017). A chemical genetic roadmap to improved tomato flavor. Science , 355 , 391–394.
- Tomato Genome Consortium . (2012). The tomato genome sequence provides insights into fleshy fruit evolution. Nature , 485 , 635–641.
- Upadhyay, R. K. , & Mattoo, A. K. (2018). Genome-wide identification of tomato ( Solanum lycopersicum L.) lipoxygenases coupled with expression profiles during plant development and in response to methyl-jasmonate and wounding. Journal of Plant Physiology , 231 , 318–328.
- USDA . (2019). Food Data Central . USDA Agricultural Research Service, Beltsville.
- Valero, D. , & Valero, M. (2013). Growth and ripening stage at harvest modulates postharvest quality and bioactive compounds with antioxidant activity. Stewart Postharvest Review , 3 , 7.
- van der Knaap, E. , Chakrabarti, M. , Chu, Y. H. , Clevenger, J. P. , Illa Berenguer, E. , Huang, Z. , . . . Wu, S. (2014). What lies beyond the eye: The molecular mechanisms regulating tomato fruit weight and shape. Frontiers in Plant Science , 5 , 227.
- Vannini, C. , Campa, M. , Iriti, M. , Genga, A. , Faoro, F. , Carravieri, S. , . . . Bracale, M. (2007). Evaluation of transgenic tomato plants ectopically expressing the rice Osmyb4 gene. Plant Science , 173 , 231–239.
- Vinocur, B. , & Altman, A. (2005). Recent advances in engineering plant tolerance to abiotic stress: Achievements and limitations. Current Opinion in Biotechnology , 16 , 123–132.
- Virtanen, J. K. , Voutilainen, S. , Nurmi, T. , Tuomainen, T.-P. , & Mursu, J. (2013). Intake of fruit, berries, and vegetables and risk of type 2 diabetes in Finnish men: The Kuopio Ischaemic Heart Disease Risk Factor Study. The American Journal of Clinical Nutrition , 99 , 328–333.
- Vrebalov, J. , Pan, I. L. , Arroyo, A. J. M. , McQuinn, R. , Chung, M. , Poole, M. , . . . Irish, V. F. (2009). Fleshy fruit expansion and ripening are regulated by the tomato SHATTERPROOF gene TAGL1 . The Plant Cell , 21 , 3041–3062.
- Waller, J. C. , Akhtar, T. A. , Lara-Nunez, A. , Gregory, J. F. , McQuinn, R. P. , Giovannoni. J. J. , . . . Hanson, A. D. (2010). Developmental and feedforward control of the expression of folate biosynthesis genes in tomato fruit. Molecular Plant , 1 , 66–77.
- Wang, B. Q. , Zhang, Q. F. , Liu, J. H. , & Li, G. H. (2011). Overexpression of PtADC confers enhanced dehydratation and drought tolerance in transgenic tobacco and tomato: Effect on ROS elimination. Biochemical and Biophysical Research Communications , 413 , 10–16.
- Wang, H. S. , Yu, C. , Tang, X. F. , Zhu, Z. J. , Ma, N. N. , & Meng, Q. W. (2014). A tomato endoplasmic reticulum (ER)-type omega-3 fatty acid desaturase (LeFAD3) functions in early seedling tolerance to salinity stress. Plant Cell Reports , 33 , 131–142.
- Wang L.-Y. , Li D. , Deng Y.-S. , Lv W. , & Meng Q.-W. (2013). Antisense-mediated depletion of tomato GDP-l-galactose phosphorylase increases susceptibility to chilling stress . Journal of Plant Physiology , 170 , 303–314.
- Wang, Y. , Wang, H. , Ma, Y. , Du, H. , Yang, Q. , & Yu, D. (2015). Identification of transcriptional regulatory nodes in soybean defense networks using transient co-transactivation assays . Frontiers in Plant Science , 6 , 915.
- Wang, Y. , Wisniewski, M. , Meilan, R. , Cui, M. , & Fuchigami, L. (2006). Transgenic tomato ( Lycopersicon esculentum ) overexpressing cAPX exhibits enhanced tolerance to UV-B and heat stress. Journal of Applied Horticulture , 8 , 87–90.
- Wasternack, C. , Stenzel, I. , Hause, B. , Hause, G. , Kutter, C. , Maucher, H. , . . . Miersch, O. (2006). The wound response in tomato—role of jasmonic acid. Journal of Plant Physiology , 163 , 297–306.
- Xu, C. , Park, S. J. , Van Eck, J. , & Lippman, Z. B. (2016). Control of inflorescence architecture in tomato by BTB/POZ transcriptional regulators. Genes & Development , 30 , 2048–2061.
- Xu, J. , van Herwijnen, Z. O. , Dräger, D. B. , Sui, C. , Haring, M. A. , & Schuurink, R. C. (2018). SlMYC1 regulates type VI glandular trichome formation and terpene biosynthesis in tomato glandular cells. The Plant Cell , 30 , 2988–3005.
- Xu, X. , Fang, P. , Zhang, H. , Chi, C. , Song, L. , Xia, X. , . . . Yu, J. (2019). Strigolactones positively regulate defense against root-knot nematodes in tomato. Journal of Experimental Botany , 70 (4), 1325–1337.
- Yamada, T. , Hayasaka, S. , Shibata, Y. , Ojima, T. , Saegusa, T. , Gotoh, T. , . . . Jichi Medical School Cohort Study Group . (2011). Frequency of citrus fruit intake is associated with the incidence of cardiovascular disease: The Jichi Medical School cohort study. Journal of Epidemiology , 21 , 169–175.
- Yang, J. Y. , Sun, Y. , Sun, A. Q. , Yi, S. Y. , Qin, J. , Li, M. H. , . . . Liu, J. (2006). The involvement of chloroplast HSP100/ClpB in the acquired thermotolerance in tomato. Plant Molecular Biology , 62 , 385–395.
- Yarra, R. , He, S. J. , Abbagani, S. , Ma, B. , Bulle, M. , & Zhang, W. K. (2012). Overexpression of wheat Na+/H+ antiporter gene ( TaNHX2 ) enhances tolerance to salt stress in transgenic tomato plants ( Solanum lycopersicum L.). Plant Cell, Tissue and Organ Culture , 111 , 49–57.
- Yazdani, M. , Sun, Z. , Yuan, H. , Zeng, S. , Thannhauser, T. W. , Vrebalov, J. , . . . Li, L. (2019). Ectopic expression of ORANGE promotes carotenoid accumulation and fruit development in tomato. Plant Biotechnology , 17 (1), 33–49.
- Zhang, C. , Liu, J. , Zhang, Y. , Cai, X. , Gong, P. , Zhang, J. , . . . Ye, Z. (2011). Overexpression of SlGMEs leads to ascorbate accumulation with enhanced oxidative stress, cold, and salt tolerance in tomato. Plant Cell Reports , 30 , 389–398.
- Zhang, M. , Yuan, B. , & Leng, P. (2009). The role of ABA in triggering ethylene biosynthesis and ripening of tomato fruit. Journal of Experimental Botany , 60 , 1579–1588.
- Zhang, Y. , Butelli, E. , Alseekh, S. , Tohge, T. , Rallapalli, G. , Luo, J. , . . . Martin, C. (2015). Multi-level engineering facilitates the production of phenylpropanoid compounds in tomato. Nature Communications , 6 , 8635.
- Zhong, S. L. , Fei, Z. J. , Chen, Y. R. , Zheng, Y. , Huang, M. Y. , Vrebalov, J. , . . . Giovannoni, J. J. (2013). Single-base resolution methylomes of tomato fruit development reveal epigenome modifications associated with ripening. Nature Biotechnology , 31 , 154–159.
- Zhou, Y. , Cho, W. K. , Byun, H.-S. , Chavan, V. , Kil, E.-J. , Lee, S. , & Hong, S.-W. (2019). Genome-wide identification of long non-coding RNAs in tomato plants irradiated by neutrons followed by infection with Tomato yellow leaf curl virus. PeerJ , 7 , e6286.
- Zhu, J.-K. (2009). Active DNA demethylation mediated by DNA glycosylases. Annual Review of Genetics , 43 , 143–166.
Printed from Oxford Research Encyclopedias, Environmental Science. Under the terms of the licence agreement, an individual user may print out a single article for personal use (for details see Privacy Policy and Legal Notice).
date: 20 April 2024
- Cookie Policy
- Privacy Policy
- Legal Notice
- Accessibility
- [66.249.64.20|81.177.182.154]
- 81.177.182.154
Character limit 500 /500
Thank you for visiting nature.com. You are using a browser version with limited support for CSS. To obtain the best experience, we recommend you use a more up to date browser (or turn off compatibility mode in Internet Explorer). In the meantime, to ensure continued support, we are displaying the site without styles and JavaScript.
- View all journals
- My Account Login
- Explore content
- About the journal
- Publish with us
- Sign up for alerts
- Open access
- Published: 16 July 2022
Organic and in-organic fertilizers effects on the performance of tomato ( Solanum lycopersicum ) and cucumber ( Cucumis sativus ) grown on soilless medium
- Aruna Olasekan Adekiya 1 , 4 ,
- Samuel Olatunde Dahunsi 2 ,
- Jerry Femi Ayeni 3 ,
- Charity Aremu 1 ,
- Christopher Muyiwa Aboyeji 1 ,
- Faith Okunlola 1 &
- Adeoluwa Emmanuel Oyelami 1
Scientific Reports volume 12 , Article number: 12212 ( 2022 ) Cite this article
14k Accesses
11 Citations
8 Altmetric
Metrics details
- Environmental sciences
- Plant sciences
The effects of organic fertilizers, based on Tithonia diversifolia , and in-organic fertilizers, based on hydroponics fertilizer, were evaluated on the performance, leaves, and fruit mineral concentrations of tomato ( Solanum lycopersicum ) and cucumber ( Cucumis sativus ) plants grown under soilless medium in a screen house. The treatments comprised six levels of liquid organic fertilizer (5, 15, 25, 35, 45, 55 mL), in-organic fertilizer, and a control. Both organic and in-organic fertilizers increased the growth, yield, leaf nutrient concentration, and the mineral contents of tomato and cucumber fruits in comparison with the control. In-organic fertilizer enhanced the performance and mineral concentrations of tomato and cucumber fruits in comparison with organic fertilizer. However, leaf analyses showed that all the essential elements for both tomato and cucumber crops were within the adequate ranges in the organic fertilizer treatments suggesting that this organic fertilizer can be used as an alternative to the expensive and scarce in-organic fertilizer. For organic fertilizer, the highest yield and mineral contents in fruits were attained at doses of 35 mL and 25 mL for tomato and cucumber plants, respectively. At these doses, the fruit weights of tomato and cucumber were increased by 137 and 198%, respectively, in comparison with the control. For a good yield of tomato and cucumber crop with a high fruit mineral content under the soilless medium of coco peat and rice husk, 35 mL and 25 mL of our tested organic fertilizer are recommended.
Similar content being viewed by others
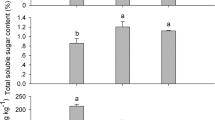
Bio-organic fertilizer with reduced rates of chemical fertilization improves soil fertility and enhances tomato yield and quality
Lin Ye, Xia Zhao, … Kai Cao
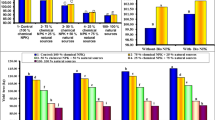
Partially substituting chemical NPK fertilizers and their impact on Eureka lemon trees (Citrus limon L. Burm) productivity and fruit quality
Abdulrhman A. Almadiy, Ayman E. Shaban, … El-Sayed G. Khater
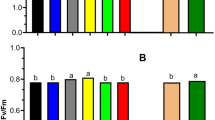
Foliar application of pyroligneous acid acts synergistically with fertilizer to improve the productivity and phytochemical properties of greenhouse-grown tomato
Raphael Ofoe, Seyed Mohammad Nasir Mousavi, … Lord Abbey
Introduction
Today, Nigeria's population stands at 200 million and is projected to be about 401 million by the year 2050 1 . Sub- Saharan African countries are faced with diverse food challenges 2 . To be able to feed the growing population adequately, food production should approximately double its present output. One of the major constraints to food crop production in the tropics is soil-related problems 3 . In Nigeria, the largest soil order—Alfisol—experiences many unfavorable challenges such as inherently low fertility, soil acidity, poor structure and high susceptibility to crusting, compaction, accelerated erosion, and a decrease in natural resources, among other things, soil diversity reduction 4 . Also, as a result of climate change, the issue of desertification and shorter rainy seasons compounds the problem of food insecurity. Furthermore, growing crops is somewhat difficult in soil-open field agriculture because they require larger areas, many workers, and a large volume of water 5 . Therefore, cropping techniques that bring good control over the environment during the cultivation of a crop give good growth and development of it 6 .
Soilless farming is a cultivation technique by which crops are grown detached from the soil. Crops are grown in a container filled with several possible growing media with nutrients supplied. Soilless cultivation is intensively used in protected agriculture especially for crops during months when field production is not possible, to improve control of the growing environment, and to avoid uncertainties in the soil's water and nutrient status 7 . They are considered important technologies for better water use efficiency as well as high quality and quantity products and they are well adapted to growing vegetable crops such as tomato and cucumber.
Tomato ( Solanum lycopersicum L.) is a vegetable crop that is cultivated all over the world and is a good source of vitamins A, B6, C, K, and E. It also contains molybdenum, copper, potassium, manganese, and is a good source of dietary fiber. The production of tomatoes using soilless techniques has been reported by several workers 8 , 9 and cucumber ( Cucumis sativus L.) is an important vegetable due to its use as a popular fresh market vegetable in salads and it is one of the most popular members of the Cucurbitaceae family 10 . Cucumbers contain phytonutrients such as flavonoids, lignans, and triterpenes, which have antioxidants, and anti-inflammatory and anti-cancer benefits. The seeds are also a good source of minerals 11 .
The successful production of tomato and cucumber fruits requires an increase in both yield and fruit quality.
The effects of organic and in-organic nutrient sources in soilless techniques on the growth and quality parameters of vegetables had been carried out by many researchers 14 . Phibunwatthanawong and Riddech 15 found that liquid organic fertilizer had similar growth promotion properties as in-organic fertilizer in the growth of green cos lettuce ( Lactuca sativa var. longifolia ). Also, studies on the effects of organic nutrient sources on field vegetable production had been reported 3 , 16 . However, no research studies have been done on soilless vegetable production in Nigeria with the use of either organic or in-organic nutrient sources. Liedl et al. 17 found that liquid effluent of digested poultry litter appeared to function as well as a commercial hydroponic fertilizer for tomatoes after balancing the forms of N (NO 3 /NH 4 ) and supplementing with Ca(NO 3 ) 2 and MgSO 4 . In Nigeria, no such study existed for soilless cropping.
One of the major ingredients of soilless cropping is inorganic nutrients. The use of inorganic chemical nutrients for agriculture is relatively expensive worldwide 12 . There is an increasing number of screen house farmers who are worried about the cost of hydroponics fertilizers in Nigeria where the rate of exchange of Nigeria Naira to US Dollar is too high and therefore the return rate or benefit/cost ratio of using hydroponics fertilizer is too low. These concerns have started a search for local materials that are readily available (grows along major roads, paths, and on abandoned farmlands in almost every agro-ecological zones of Nigeria) and affordable which could be used as an alternative such as fertilizer obtained from the biomass of Mexican sunflower ( Tithonia diversifolia ).
In addition, during the last decades, the quest for organic products has risen because many people are environmentally conscious and believe that organic products are healthier than conventional products 13 .
Although soilless cropping is done in a controlled environment, reactions of crops in terms of yield and quality of crop to organic nutrients may still be subjected to the environment (tropics, subtropics, or temperate), the quality (chemical properties of different organic material), type of material used and methods for producing organic materials used as organic liquid fertilizer. These aspects need investigation. Therefore, this study was conducted to investigate the effects of organic and inorganic nutrient sources on fruit yield, growth, leaf nutrient concentration, and fruit mineral contents of tomato and cucumber plants grown under the soilless medium.
Effects of organic and in-organic fertilizers on growth and yield of tomato and cucumber plants under soilless medium
Figures 1 and 2 , and Table 1 , respectively, show the results of the effects of organic and in-organic fertilizers on the number of fruits, fruit weight, and growth parameters of tomato and cucumber plants under soilless medium. Both organic and in-organic fertilizers increased the growth (plant height, number of leaves, leaf area, and stem diameter) (Table 1 ) and the yield (number of fruits and fruit weight) (Figs. 1 and 2 ) of tomato and cucumber plants significantly compared with the control. For both tomato and cucumber crops, in-organic fertilizer significantly increased growth and yield compared with the organic fertilizer at any dose. Average from both site a and site B, for tomato, in-organic fertilizer increased the yield by 99.04, 76.5, 57.2, 41.1, 49.4, 49.3, and 298.0%, respectively for 5 mL, 15 mL, 25 mL, 35 mL, 45 mL, 55 mL, and the control. Likewise for cucumber, using the average from the two sites, in-organic fertilizer increased the fruit yield of cucumber by 84.8, 46.0, 24.2, 48.01, 56.1, 56.8, and 497.1% respectively for 5 mL, 15 mL, 25 mL, 35 mL, 45 mL, 55 mL, and the control. Also, organic fertilizer increased the growth (plant height, number of leaves, leaf area, and stem diameter) of the two crops up to 55 mL dose.
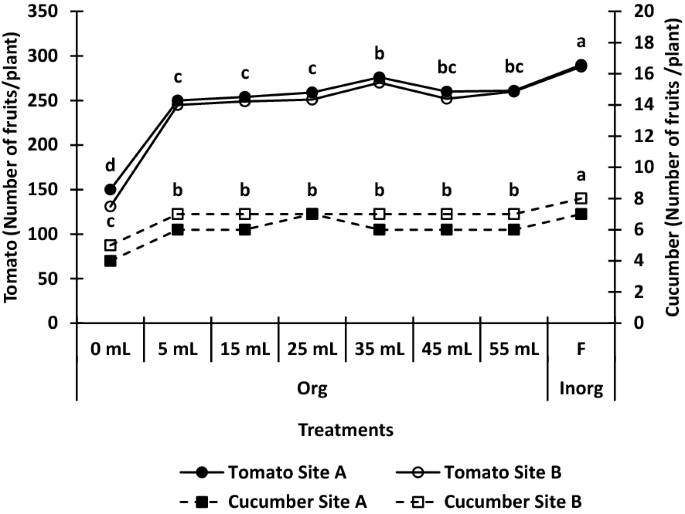
Effect of organic and in-organic fertilizers on number of fruits of tomato and cucumber under soilless medium. Values followed by similar letters under the same graph are not significantly different at p = 0.05 according to Duncan’s multiple range test. Org organic fertilizer, F inorg in-organic fertilizer.
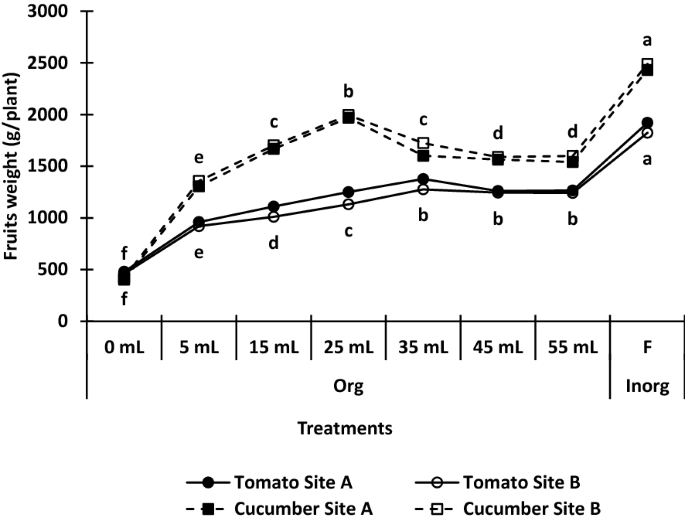
Effect of organic and in-organic fertilizers on fruit weight of tomato and cucumber under soilless medium. Values followed by similar letters under the same graph are not significantly different at p = 0.05 according to Duncan’s multiple range test. Org organic fertilizer, F inorg in-organic fertilizer.
At site A for tomato, 55 mL organic fertilizer increased plant height, number of leaves, leaf area and stem girth relative to the control by 356.0, 97.0, 91.4, and 70.3% respectively, while for site B relative to the control it was 326.9, 86.1, 94.4 and 69.2%, respectively for plant height, number of leaves, leaf area, and stem girth. Also, At site A for cucumber, 55 mL organic fertilizer increased plant height, number of leaves, leaf area and stem girth relative to the control by 277.3, 190.5, 95.4 and 96.0% respectively, while for site B relative to the control it was 276.6, 195.5, 98.1 and 100%, respectively for plant height, number of leaves, leaf area and stem girth. For tomato, organic fertilizer increased yield up to 35 mL level after which there was a decrease. In the same vein, organic fertilizer increased the yield of cucumber up to 25 mL level. At 35 mL for tomato and 25 mL for cucumber), the fruit weight of tomato and cucumber were increased by 137 and 198%, respectively compared with the control. Similarly, inorganic fertilizer increased the fruit weight of tomato and cucumber by 19 and 24%, respectively compared with an organic fertilizer at their optimum level.
Effects of organic and in-organic fertilizers on leaf nutrient concentrations of tomato and cucumber under soilless medium
Data on the effects of organic and inorganic fertilizers on leaf nutrient concentrations of tomato and cucumber are presented in Table 2 . For both tomato and cucumber leaves, nutrient concentration was significantly higher in organic and in-organic treatments compared with the control. Inorganic fertilizer has higher values of N, P, K, Ca, and Mg in tomato leaves compared with organic fertilizer. Organic fertilizer increased nutrient concentration in tomato leaves up to 35 mL level after which there was a decrease at 45 and 55 mL levels.
For cucumber, leaf N, P, K, Ca, and Mg with organic fertilizer were increased from 5 to 25 mL application level after which there was a reduction. There were no significant differences between 5 mL, 55 mL, and inorganic fertilizer for N and K in cucumber, also all levels of organic fertilizer produced significantly higher values of P and Mg in cucumber leaves compared with inorganic fertilizer except those for 45 and 55 mL levels.
Effects of organic and in-organic fertilizers on mineral contents of tomato and cucumber fruits under soilless medium
Results of the effects of organic and inorganic fertilizers on mineral contents of tomato and cucumber fruits under soilless conditions are presented in Table 3 . In both tomato and cucumber crops, mineral contents of the fruits were significantly higher under organic and inorganic fertilizers compared with the control. Mineral contents were also higher in inorganic treatment compared with organic treatments. Tomato has significantly higher mineral content (P, K, Ca, Mg and Fe) at 35 mL level of organic fertilizer. Similarly, cucumber has its optimum mineral nutrient concentration at 25 mL of organic fertilizer. The value of N in both crops increased by up to 55 mL.
The increase in growth and yield of tomato and cucumber crops in the present study due to the application of organic and inorganic fertilizers was a result of the increase in nutrient status of the substrate because of the applied nutrients leading to absorption, hence significant improvement in growth, yield leaf nutrient concentration and fruit mineral levels of tomato and cucumber crops.
The results are in agreement with that of Mowa et al. 18 that organic plant sources can certainly supply adequate nutrients for good growth and yield of crops under soilless cropping. The improved growth as the rates of organic fertilizer increase can be adduced to increased nutrient supply, especially N that is needed for vegetable growth of crops.
For both tomato and cucumber crops, the number of fruits per plant was higher in inorganic fertilizer compared with organic fertilizers. Tonfack et al. 19 had earlier reported an increase in fruit per plant where in-organic fertilizer was used relative to organic fertilization.
Organic fertilizer reduced the growth, yield, and mineral contents of tomato and cucumber fruits compared with inorganic fertilizer. This was due to low availability, slow release, and low uptake of organic nutrients by the crops compared with the inorganic fertilizer. Mowa et al. 18 reported that organic fertilizers are unsuitable for planting growth because N in organic fertilizers is predominantly organic, hence unusable by plants. Unlike inorganic fertilizer that releases nutrients upon application/fertigation, organic fertilizer has to mineralize first before being available to crops. Baghdadi et al. 20 reported that the number of nutrients which contacts directly with the plant roots in organic fertilized crops is rather small within the overall nutrient demand hence reducing growth and yield compared with inorganic fertilizer. Another reason for the better growth and yield of crops under inorganic compared to organic fertilizer was due to differences in nitrate and other nutrient levels between the inorganic nutrient solution and organic nutrient solution.
In the current study, inorganic fertilizer had NH 4 -N (48.7 mg L −1 N), NO 3 -N (173.4 mg L −1 N), PO 4 -P (107.8 mg L −1 P), K (173.8 mg L −1 ), Ca (158.5 mg L −1 ), Mg (80 mg L −1 ) and SO 4 -S (106.7 mg L −1 S) compared with organic nutrient with 48.5, 3.41, 4.5, 8.0, 100.0 and 84.0 mg L −1 respectively for N, P, K, Ca, Mg and SO 4 (Table 4 ). Likewise, results showed that growth parameters of tomato and cucumber increased with the increase of organic nutrient levels up to 55 mL level. In contrast, the yield was increased with tomato and cucumber up to 35, and 25 mL respectively can testify that in the experimental condition, the 35 mL was over optimal for the yield of fruits of tomato and 25 mL was for cucumber fruits. The results corroborated that of Simpson 21 who found that tomato fruit yields were depressed as N rate was increased by producing excessive luxuriant vegetative parts of the plant at the expense of reproductive growth. Similarly, excessive fertilization of N fertilizer has been reported to cause decreases in the commercial yield of cucumber 22 . Therefore, Nitrogen fertilization must be carefully managed to attain a high marketable yields while minimizing the adverse effects of excessive vine growth. For organic fertilizer, this high marketable yield was attained for tomato at 35 mL and 25 mL for cucumber. It shows that tomato requires more nutrients than cucumber. This fact is evident in the higher nutrient concentrations of tomatoes compared to that of cucumbers (Table 2 ).
Leaf nutrient concentration was significantly higher in organic and inorganic treatments compared with the control. The response of leaf nutrient concentrations of tomato and cucumber leaves to organic and inorganic fertilizer was consistent with the values of nutrients recorded for organic fertilizer (Table 4 ) and that for inorganic fertilizer. There was increased nutrient availability in the substrate leading to increased uptake by tomato and cucumber plants. Leaf nutrient concentrations of inorganic fertilizer under tomato crops were improved compared with those of the organic fertilizers because of the quick and easy absorption of nutrients in the inorganic treatments. In cucumber crops, organic fertilizer improved leaf nutrient concentration compared with inorganic fertilizer. This was due to the fact that some of the nutrient elements may have been converted to assimilate (fruits). In the present experiment, inorganic fertilizer has a higher yield compared with organic fertilizer for cucumber. Furthermore, for this experiment, leaf analyses showed that all the essential elements for both tomato and cucumber crops were within the adequate ranges in the organic and inorganic fertilizer treatments as suggested by 23 for greenhouse-grown tomato (4.5% N, 0.56% P, 5.72% K, 4.4% Ca and 0.50% Mg) and de Kreij et al. 24 for cucumber (4.2% N, 0.6 P, 3.2% K, 2.2 Ca and 0.40% Mg) at optimum levels. It shows that the amounts of other nutrients required for tomato plant growth were sufficient in the organic nutrient solution to result in similar growth patterns as those from the inorganic fertilizer. In this experiment, the use of Tithonia biomass as fertilizer in tomato and cucumber production was carried out with virtually no cost relative to the expensive inorganic fertilizer. It suggested that this organic fertilizer can be used as an alternative for the expensive and scarce inorganic fertilizer in developing countries like Nigeria. Chang et al. 25 also found that organic fertilizer produced from pea and rice hull compost can successfully replace chemical fertilizer for cut flower production of Anthurium andreanum .
Except for N, the mineral contents of both tomato and cucumber fruits increased up to 35 mL and 25 mL respectively, due to nutrient imbalance after the optimum level of nutrients 3 . At a high N rate from the excessive rate of nutrients, crops (tomato and cucumber) produced many leaves with dark green flourishing big growth and abnormal cell as a result of lack of other elements such as Mg, K, and Ca. The fruits have high water content but fewer flavors and become watery 3 . Roper 26 reported that nutrients in the fruits declined with an increase in nutrient applications.
The results of this study revealed that both organic and inorganic fertilizers increased the growth, yield, leaf nutrient concentration, and the mineral contents of tomato and cucumber fruits compared with the control. Although organic fertilizer reduced the growth, yield, and mineral contents of tomato and cucumber fruits compared with inorganic fertilizer (which was due to low availability, slow-release, and low uptake of organic nutrients by the crops compared with the inorganic fertilizer), leaf analyses showed that all the essential elements for both tomato and cucumber crops were within the adequate ranges in the organic fertilizer treatments suggesting that this organic fertilizer can be used as an alternative for the expensive and scarce inorganic fertilizer. Also, for organic fertilizer in this experiment, the highest yield and mineral contents were attained for a tomato at 35 mL and 25 mL for cucumber. It shows that this is the optimum level for these crops, and that tomato requires more nutrients than cucumber. Therefore, for a good yield of tomato and cucumber with high mineral content under the soilless medium of coco peat and rice husk, 35 mL and 25 mL are recommended for tomato and cucumber respectively.
Material and methods
Growth conditions and plant materials.
Two experiments were conducted concurrently (sites A and B) in the same screen house in 2019 between the months of May and July at the Landmark University Greenhouse and Hydroponic Technology Center, a section of the Teaching and Research Farm of the University in Omu-Aran, Kwara State Nigeria. Experiment at site B was conducted simultaneously as A so as to validate the results of experiment A. Landmark University lies within Latitude 8° 7′ 26.21388″ and 5° 5′ 0.1788″. Both experiments (A & B) involved tomato ( Solanum lycopersicum L. variety cherry) and cucumber ( Cucumis sativus L. variety marketer) crops. For each crop, seeds were sown into a separate seed tray filled with coco peat (Coco peat, SRIMATHI EXPORT, INDIA). Cocopeat is the mesocarp tissue or husk after the grinding of coconut fruit. It has a lightweight and high water and nutrient holding capacities, it has an acceptable pH, electrical conductivity, and other chemical attributes 27 . Rice husk is the by-product of rice after milling. The rice husk used was collected from the rice processing mill of Landmark University. Rice husk is a highly porous and light weighted material with a very high specific area 28 .
Two sets of seed trays (one for organic and another for inorganic fertilizers) were used each for tomato and cucumber crops in the nursery. Both were raised in the nursery for two weeks before transplanting. Black grow bags (30 × 17 cm) filled with a coco peat/rice husk (1:4 ratio by volume) mixture with a weight of about 10 kg were arranged in a screen house. Both the nursery and establishment of crop proper take place in a screen house. The screen house has a galvanized iron as the frame, a UV covering on top, side net for screening insect pests the floor fairly covered with granite. Temperature and relative humidity within the screen house during the period of the experiment was monitored using a Thermograph and a Barograph, and they were at an average of 31 °C and 75%, respectively.
The grow bags were randomly placed in the screen house for the unbiased application of amendments. For both tomato and cucumber crops, the treatment comprised of six (6) levels of liquid organic fertilizer (5, 15, 25, 35, 45, 55 mL), in-organic fertilizer, and a control (ordinary borehole water). Levels of organic fertilizers were selected based on the recommendation of 20 mL of liquid organic fertilizer by 29 . The eight (8) treatments both for tomato and cucumber were arranged in a Completely Randomized Design replicated three times. One healthy plant was maintained per grow bag and four grow bags represent a treatment and there were 32 plants per block each for tomato and cucumber. For both crops, the experiment lasted for 90 days.
Organic and in-organic nutrient solutions
The liquid organic fertilizer used was obtained from the biomass of Mexican sunflower ( Tithonia diversifolia ). Fresh biomass (mainly leaves and stems) of the plant was collected from the Teaching and Research Farms of Landmark University, Nigeria. After rinsing, they were cut with a sterile knife into pieces of ≤ 1 cm size. A sample was taken for initial physicochemical analyses by grinding in a sterile mortal, diluted with sterile water and analyzed. The biomass was then soaked in sterile water inside a clean container, and allowed to ferment spontaneously for a period of 14 days. During the fermentation, samples were taken every 4 days for microbial analyses of the major players during the fermentation. At the end of fermentation, the mixture was separated using a sieve of mesh size ≤ 2 mm. The liquid portion was then refrigerated prior to the planting regime while another sample was taken to ascertain the physicochemical and microbial qualities of the produced liquid fertilizer. The chemical analysis is presented in Table 4 . For inorganic fertilizer, Water soluble fertilizers employed in hydroponics were used (Hydroponics fertilizer, Anmol chemicals, India); calcium nitrate 650 mg L −1 , potassium nitrate 450 mg L −1 , magnesium 400 mg L −1 , chelate 20 mg L −1 , mono-ammonium phosphate 400 mg L −1 . The electrical conductivity (EC) of the solution was 1.9 dS m -1 .
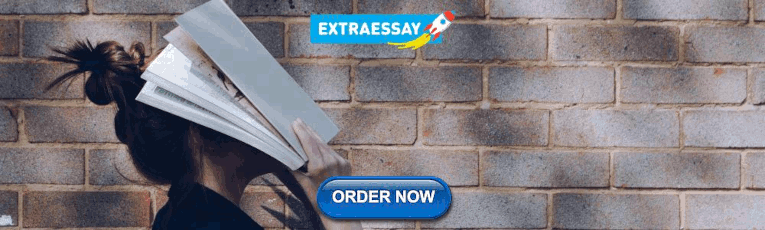
Irrigation and fertigation
The tomato and cucumber plants were fertigated morning and evening daily for one hour on each occasion according to the treatments. Preparation of the nutrient solution was with borehole water and was supplied to plants by an online pressure drip irrigation system set at 2.0 L h -1 using an arrowhead on each tomato and cucumber plant. Different tanks (250 L) were installed according to the various treatments making a total of 8 tanks. The organic fertilizer was diluted according to the various treatments equivalent to 1.25, 3.75, 6.25, 8.75, 11.25, and 13.75 L per 250 L of water respectively for 5, 15, 25, 35, 45, and 55 mL treatments. The nutrient solutions were refilled when the consumption is less than 20% of the initial volume (250 L) in the tank. One day per week, crops were irrigated with ordinary water to wash out pipes and prevent deposits of salts. The same concentration of nutrient was used from transplanting to the termination of the study for both tomato and cucumber crops, however, at the flowering of the crops, the volume of fertigation was increased to 3.0 L h -1 to be able to cope with the size of the plants.
Trellising, pest and diseases control
For both tomato and cucumber crops, plant vines were supported by twisting them around a wire that is- attached to the roof of the screen house and 2 m from the ground. Lateral outgrowths were cut off every week to ensure a sturdy single stem. Pests and diseases were scouted every day. Whiteflies, aphids, and other insects were controlled with orizon (Producer, location of producer) (active ingredient, acetamiprid, and abamectin) using 0.133% v/v. Fungi were controlled using ridomil gold (Producer, Location of producer) at 2% w/v.
Determination of growth and yield of tomato and cucumber
Three tomato and cucumber plants were randomly selected for each treatment for the determination of growth parameters (plant height, leaf area, number of leaves per plant, and stem diameter) at mid the flowering stage of tomato and cucumber plants.The leaf area of tomato was calculated using the model (A = KL2) developed by Lyon 30 , where L = Length of tomato leaf, K = constant which is 0.1551, and A = leaf area of tomato. Similarly, the leaf area of cucumber was calculated using A = 0.88LW – 4.27, where L = cucumber leaf length and W = cucumber leaf width, A = leaf area of cucumber 31 .
Tomato fruits were ready for harvest from 65 days after transplanting, harvestings were done twice every week (Mondays and Fridays) for up to 85 days after transplanting. Similarly, harvesting of cucumber fruits started 35 days after transplanting and harvestings were also done twice a week (Mondays and Fridays), harvesting was carried out till 60 days after transplanting. Tomato and cucumber fruit yields were counted and weighed at each harvest.
Analysis of tomato and cucumber leaves and fruits
At the 50% flowering stage of tomato and cucumber plants, ten leaf samples were collected from each treatment. The leaf samples were oven-dried at 75 °C for 24 h and thereafter grounded. The grounded samples were later analyzed for nitrogen (N), phosphorous (P), potassium (K), calcium (Ca), and magnesium (Mg) content using the method of described by 32 . At harvest, four matured tomato and cucumber fruits of uniform size were selected per treatment, and their nutrient compositions were determined using the method of 33 .
Statistical analysis
All data collected on the growth, yield, leaf, and fruit nutrient contents of tomato and cucumber were subjected to analysis of variance (ANOVA). The SPSS V 21.0 (New York, USA) software was used to perform ANOVA and Duncan’s multiple range test (DMRT) was used to compare means at a 5% probability level.
Ethical approval
I confirm that all the research meets ethical guidelines and adheres to the legal requirements of the study country.
Compliance with international, national and/or institutional guidelines
Experimental research (either cultivated or wild), comply with relevant institutional, national, and international guidelines and legislation. Experimental studies were carried out in accordance with relevant institutional, national or international guidelines or regulation.
Data availability
All datasets generated and/or analyzed during the current study are included in this article.
UN. World Urbanization Prospects (United Nations, 2018).
Google Scholar
OECD/Food and Agriculture Organization of the United Nations Agriculture in Sub-Saharan Africa: Prospects and challenges for the next decade. In OECD-FAO Agricultural Outlook 2016–2025 (OECD Publishing, 2016). https://doi.org/10.1787/agr_outlook-2016-5-en .
Chapter Google Scholar
Adekiya, A. O. et al. Poultry manure addition affects production, plant nutritional status and heavy metals accumulation in green Amaranth ( Amaranthus hybridus ). Int. J. Agric. Biol. 22 (5), 993–1000 (2019).
CAS Google Scholar
Adekiya, A. O. & Agbede, T. M. Growth and yields of tomato ( Lycopersicon esculentum Mill) as influenced by poultry manure and NPK fertilizer. Emirate J. Food Agric. 21 (1), 10–20 (2009).
Article Google Scholar
Raza, A. et al. Impact of climate change on crops adaptation and strategies to tackle its outcome: A review. Plants 8 (34), 1–29. https://doi.org/10.3390/plants8020034 (2019).
Article CAS Google Scholar
Suvo, T. P., Biswas, H., Jewel, M. H., Islam, M. S. & Khan, M. S. I. Impact of substrate on soilless tomato cultivation. Int. J. Agril. Res. Innov. Tech. 6 (2), 82–86 (2016).
Roudsari, O. N., Peyvast, G. H., Alimosavi, A., Kharazi, P. R. & Olfati, J. A. Feasibility decrease use of fertilizer on greenhouse cucumber production in soilless culture. Biosci. Biotechnol. Res. Asia 5 (2), 593–600 (2008).
Rodríguez-Ortega, W. M. et al. Agricultural and physiological responses of tomato plants grown in different soilless culture systems with saline water under greenhouse conditions. Sci. Rep. 9 , 6733. https://doi.org/10.1038/s41598-019-42805-7 (2019).
Article ADS CAS PubMed PubMed Central Google Scholar
Bergstrand, K.-J., Löfkvist, K. & Asp, H. Dynamics of nutrient availability in tomato production with organic fertilisers. Biol. Agric. Hortic. 36 (3), 200–212. https://doi.org/10.1080/01448765.2020.1779816 (2020).
Ajuru, M. & Nmom, F. A Review on the economic uses of species of Cucurbitaceae and their sustainability in Nigeria. Am. J. Plant Biol. 2 (1), 17–24. https://doi.org/10.11648/j.ajpb.20170201.14 (2017).
Aderinola, T. A. & Abaire, K. E. Quality acceptability, nutritional composition and antioxidant properties of carrot-cucumber juice. Beverages 5 , 15. https://doi.org/10.3390/beverages5010015 (2019).
Timsina, J. Can organic sources of nutrients increase crop yields to meet global food demand? Review. Agronomy 8 , 214. https://doi.org/10.3390/agronomy8100214 (2018).
Varanasi, A. Is organic food really better for the environment? columbia climate school. Climate, Earth, and Society . https://news.climate.columbia.edu/2019/10/22/organic-food-better-environment/ . Accessed 20 April 2021.
Ortiz, J. C. R. Nutritive solutions formulated from organic fertilizers, urban horticulture. In Necessity of the Future (eds Solankey, S. S. et al. ) (IntechOpen, 2020). https://doi.org/10.5772/intechopen.89955 . https://www.intechopen.com/books/urban-horticulture-necessity-of-the-future/nutritive-solutions-formulated-from-organic-fertilizers .
Phibunwatthanawong, T. & Riddech, N. Liquid organic fertilizer production for growing vegetables under hydroponic condition. Int. J. Recycl. Org. Waste Agric. 8 , 369–380. https://doi.org/10.1007/s40093-019-0257-7 (2019).
Aboyeji, C. M. et al. Performance and quality attributes of okra ( Abelmoschus esculentus (L.) Moench) fruits grown under soil applied Zn fertilizer, green biomass and poultry manure. Sci. Rep. 11 , 8291. https://doi.org/10.1038/s41598021-87663-4 (2021).
Article CAS PubMed PubMed Central Google Scholar
Liedl, B. E., Cummins, M., Young, A., Williams, M. L. & Chatfield, J. M. Liquid effluent from poultry waste bioremediation as a potential nutrient source for hydroponic tomato production. Acta Hort. 659 , 647–652 (2004).
Mowa, E., Akundabweni, L., Chimwamurombe, P., Oku, E. & Mupambwa, H. A. The influence of organic manure formulated from goat manure on growth and yield of tomato ( Lycopersicum esculentum ). Afr. J. Agric. Res. 12 (41), 3061–3067. https://doi.org/10.5897/AJAR2017.12657 (2017).
Tonfack, L. B. et al. Impact of organic and inorganic fertilizers on tomato vigor, yield and fruit composition under tropical andosol soil conditions. Fruits 64 (3), 167–177 (2009).
Baghdadi, A., Halim, R. A., Ghasemzadeh, A., Ramlan, M. F. & Sakimin, S. Z. Impact of organic and inorganic fertilizers on the yield and quality of silage corn intercropped with soybean. PeerJ 6 , e5280. https://doi.org/10.7717/peerj.5280 (2018).
Simpson, M. Signs of Too Much Nitrogen in Soil for Tomatoes . https://homeguides.sfgate.com/signs-much-nitrogen-soil-tomatoes-72800.html . Accessed 06 June 2020.
Li, Y., Li, J., Gao, L. & Tian, Y. Irrigation has more influence than fertilization on leaching water quality and the potential environmental risk in excessively fertilized vegetable soils. PLoS ONE 13 (9), e0204570. https://doi.org/10.1371/journal.pone.0204570 (2018).
Martinez, H. E. P., Carvalho, J. G. & Souza, R. B. Diagnose Foliar. In Recomendação Para Uso de Corretivos e Fertilizantes em Minas Gerais: 5a Aproximação (eds Ribeiro, A. C. et al. ) 144–168 (Comissão de fertilidade do solo do Estado de Minas Gerais, 1999).
de Kreij, C., Sonneveld, C., Warmenhoven, M. G. & Straver, N. A. Guide Values for Nutrient Element Contents of Vegetables and Flowers Under Glass 3rd edn. (Proefstation voor Tuinbouw onder Glas te Naaldwijkl, 1992).
Chang, K. H., Wu, R. Y., Chuang, K. C., Hsieh, T. F. & Chung, R. S. Effects of chemical and organic fertilizers on the growth, flower quality and nutrient uptake of Anthurium andreanum , cultivated for cut flower production. Sci. Hortic. 125 , 434–441 (2010).
Roper, T. Taking and interpreting soil and tissue samples. Wisc. Cranberry School 11 , 16–23 (2001).
Abad, M., Noguera, P., Puchades, R., Maquieira, A. & Noguera, V. Physico-chemical and chemical properties of some coconut dusts for use as a peat substitute for containerized ornamental plants. Biores. Technol. 82 , 241–245 (2002).
Jongpradist, P., Homtragoon, W., Sukkarak, R., Kongkitkul, W. & Jamsawang, P. Efficiency of rice husk ash as cementitious material in high-strength cement-admixed clay. Adv. Civil Eng. https://doi.org/10.1155/2018/8346319 (2018).
Sopha, G. A., Efendi, A. M. & Liferdi, L. Enhancing organic tomato yield and quality by liquid organic fertilizer. J. Agron. 19 , 106–112. https://doi.org/10.3923/ja.2020.106.112 (2020).
Lyon, C. J. A factor method for the leaf area of tomato leaves. Plant Physiol. 23 (4), 634–635 (1948).
Blanco, F. F. & Folegatti, M. V. Estimation of leaf area for greenhouse cucumber by linear measurements under salinity and grafting. Sci. Agric. 62 (4), 305–309 (2005).
Tel, D. A. & Hagarty, M. Soil and Plant Analysis. Study Guide for Agricultural Laboratory Directors and Technologists Working in Tropical Regions (International Institute of Tropical Agriculture, 1984).
AOAC. Official Methods of Analysis (Association of Official Agricultural Chemists International, 2010).
Download references
Acknowledgements
I would like to thank the management of Landmark University who provided enabling environment in carrying out the experiment.
Author information
Authors and affiliations.
College of Agricultural Sciences, Landmark University, PMB 1001, Omu-Aran, Kwara State, Nigeria
Aruna Olasekan Adekiya, Charity Aremu, Christopher Muyiwa Aboyeji, Faith Okunlola & Adeoluwa Emmanuel Oyelami
Microbiology Programme, College of Agriculture, Engineering and Science, Bowen University, Iwo, Osun State, Nigeria
Samuel Olatunde Dahunsi
Department of Agricultural & Bio-System Engineering, Landmark University, PMB 1001, Omu-Aran, Kwara State, Nigeria
Jerry Femi Ayeni
Landmark University SDG 15 (Life on Land Research Group), Omu-Aran, Nigeria
Aruna Olasekan Adekiya
You can also search for this author in PubMed Google Scholar
Contributions
A.O.A: Conceptualization, Writing—original draft, review & editing; S.O.D: Resources; J.F.A: Project administration; C.A: Software; C.M.A: Investigation; F.O: Supervision; AEO: Review.
Corresponding author
Correspondence to Aruna Olasekan Adekiya .
Ethics declarations
Competing interests.
The authors declare no competing interests.
Additional information
Publisher's note.
Springer Nature remains neutral with regard to jurisdictional claims in published maps and institutional affiliations.
Rights and permissions
Open Access This article is licensed under a Creative Commons Attribution 4.0 International License, which permits use, sharing, adaptation, distribution and reproduction in any medium or format, as long as you give appropriate credit to the original author(s) and the source, provide a link to the Creative Commons licence, and indicate if changes were made. The images or other third party material in this article are included in the article's Creative Commons licence, unless indicated otherwise in a credit line to the material. If material is not included in the article's Creative Commons licence and your intended use is not permitted by statutory regulation or exceeds the permitted use, you will need to obtain permission directly from the copyright holder. To view a copy of this licence, visit http://creativecommons.org/licenses/by/4.0/ .
Reprints and permissions
About this article
Cite this article.
Adekiya, A.O., Dahunsi, S.O., Ayeni, J.F. et al. Organic and in-organic fertilizers effects on the performance of tomato ( Solanum lycopersicum ) and cucumber ( Cucumis sativus ) grown on soilless medium. Sci Rep 12 , 12212 (2022). https://doi.org/10.1038/s41598-022-16497-5
Download citation
Received : 09 February 2022
Accepted : 11 July 2022
Published : 16 July 2022
DOI : https://doi.org/10.1038/s41598-022-16497-5
Share this article
Anyone you share the following link with will be able to read this content:
Sorry, a shareable link is not currently available for this article.
Provided by the Springer Nature SharedIt content-sharing initiative
This article is cited by
Use of tannery waste anaerobic digestate as agricultural fertilizer: an analysis of oat growth and soil fertility.
- Taysnara Simioni
- Caroline Borges Agustini
- Mariliz Gutterres
Waste and Biomass Valorization (2023)
By submitting a comment you agree to abide by our Terms and Community Guidelines . If you find something abusive or that does not comply with our terms or guidelines please flag it as inappropriate.
Quick links
- Explore articles by subject
- Guide to authors
- Editorial policies
Sign up for the Nature Briefing: Anthropocene newsletter — what matters in anthropocene research, free to your inbox weekly.

ORIGINAL RESEARCH article
Tomato diseases and pests detection based on improved yolo v3 convolutional neural network.
- Facility Horticulture Laboratory of Universities in Shandong, Weifang University of Science and Technology, Weifang, China
Tomato is affected by various diseases and pests during its growth process. If the control is not timely, it will lead to yield reduction or even crop failure. How to control the diseases and pests effectively and help the vegetable farmers to improve the yield of tomato is very important, and the most important thing is to accurately identify the diseases and insect pests. Compared with the traditional pattern recognition method, the diseases and pests recognition method based on deep learning can directly input the original image. Instead of the tedious steps such as image preprocessing, feature extraction and feature classification in the traditional method, the end-to-end structure is adopted to simplify the recognition process and solve the problem that the feature extractor designed manually is difficult to obtain the feature expression closest to the natural attribute of the object. Based on the application of deep learning object detection, not only can save time and effort, but also can achieve real-time judgment, greatly reduce the huge loss caused by diseases and pests, which has important research value and significance. Based on the latest research results of detection theory based on deep learning object detection and the characteristics of tomato diseases and pests images, this study will build the dataset of tomato diseases and pests under the real natural environment, optimize the feature layer of Yolo V3 model by using image pyramid to achieve multi-scale feature detection, improve the detection accuracy and speed of Yolo V3 model, and detect the location and category of diseases and pests of tomato accurately and quickly. Through the above research, the key technology of tomato pest image recognition in natural environment is broken through, which provides reference for intelligent recognition and engineering application of plant diseases and pests detection.
Introduction
The continuous development of economy and society has brought about global climate and environmental problems. The occurrence of diseases and insect pests seriously affects people’s life. The incidence and occurrence of plant diseases and insect pests is higher and higher and more complex ( Food and Agriculture Organization of the United Nations, ). Therefore, it is very important to study the prevention of plant diseases and insect pests, as well as the diagnosis and remedial measures of plant diseases and insect pests.
The origin of tomato is South America. Tomato is one of the important economic crops, which not only contains rich vitamins, but also can be used as fruit. In recent years, with the popularity of Western food, tomato sauce is more and more popular. The demand for tomato is increasing, and it has gradually become an important food in people’s daily life. Therefore, tomato plays an extremely important role in agricultural vegetable production and vegetable trade. As one of the most widely cultivated vegetables in the world, tomato has not only high yield, wide adaptability, but also high nutritional value. But, like other crops, tomato is affected by various diseases and pests in its growth process. Diseases include tomato virus disease, tomato nematode disease, tomato deficiency disease, tomato physiological disease, tomato bacterial disease, and tomato fungal disease. Pests mainly include leaf miner, greenhouse whitefly, Alfalfa noctuid moth, tobacco green worm, cotton bollworm, and Polyphagotarsonemus latus , etc. Usually, the occurrence of tomato diseases and pests is greatly affected by the local environment, varieties, cultivation, and management factors, so the types of tomato diseases and pests in different regions are different. China is one of the largest country in world vegetable production and export. The development of Shandong Shouguang tomato industry has a strong comparative advantage in the world. The cultivation area of facilities and open-field tomatoes has gradually increased. If improper field management can increase the probability of infection by diseases and pests, leading to outbreaks of diseases and pests, which has a significant impact on tomato yield and quality. Field investigation revealed that common tomato diseases in Shouguang, Shandong Province mainly include early blight, late blight, yellow leaf curl virus, brown spot, coal pollution, gray mold, leaf mold, navel rot, leaf curl disease, mosaic; common tomato pests mainly include leaf miner and greenhouse whitefly. According to the statistical data analysis of field investigation, it can be seen that the incidence of tomato diseases and pests in different farm households varies greatly in the field, with the yield loss of 1%–5% in the area with mild onset and more than 30% in the area with severe onset. At present, although many studies have reported the infection and detection of tomato pests and diseases in different regions, there is a lack of systematic reports on the detection of the main pests and diseases of tomatoes commonly found in Shouguang, Shandong Province. Therefore, it is very necessary to take 10 common diseases and 2 common insect pests in Shouguang area of Shandong Province as the research objects, and collect and collate the relevant data to provide theoretical basis for targeted early warning and prevention and control in the field.
The occurrence of tomato diseases and pests in different regions seriously affects tomato production. If the control is not timely, it will lead to yield reduction or even crop failure. Disease and pest prevention is the best way to reduce yield loss and reduce pesticide application to produce pollution-free vegetables. When plants grow to the point where symptoms of pests and diseases already occur, even if people can make accurate diagnosis and appropriate treatment, it is also a passive remedy. Although this is also very necessary, at this time, the application of agricultural chemicals and pesticides has poor control effect and is easy to cause environmental pollution, which leads to excessive pesticide residues in vegetables, and at the same time leads to more and more resistance of pests and diseases, making the work of crop disease resistance more and more difficult, which is an undesirable result. Therefore, early prediction and prevention of diseases and pests are very important. Research on tomatoes ( Diaz-Pendon et al., 2010 ; Gilbertson and Batuman, 2013 ) shows how susceptible a plant is to be influenced by diseases and pests. With regard to how to effectively control diseases and insect pests and help vegetable farmers to improve the yield of tomato, the most important thing is to make accurate identification of diseases and insect pests. Therefore, the identification of tomato diseases and insect pests is the most serious challenge for scientific and technical personnel.
The traditional method of artificial detection of diseases and insect pests completely depends on the observation experience of the grower, or ask experts for guidance. Such a method is not only slow, but also is of low efficiency, high cost, strong subjectivity, low accuracy, and timeliness. With the continuous development of the Internet, the application of information technology provides new methods and ideas for crop diseases and insect pests’ identification. Using efficient image recognition technology can improve the efficiency of image recognition, reduce the cost, and improve the recognition accuracy. Therefore, experts and scholars at home and abroad have done a lot of research, in which deep learning has become the research focus. The application of deep learning in crop diseases and insect pests’ identification can greatly reduce the workload and shorten the identification time. Complex network structure and huge data samples are the biggest characteristics of deep learning. The emergence of deep learning technology provides strong technical support for image recognition.
Among them, the convolutional neural network (CNN) is a typical model of deep learning. The diseases and pests detection method based on CNN can automatically extract the features in the original image, which overcomes the subjectivity and limitation of artificial feature extraction in the traditional methods. The end-to-end structure simplifies the recognition process and solves the problems that manually designed feature extractor cannot get the feature expression closest to the natural attribute of the object. Based on the application of CNN object detection, not only can save time and effort, but also can achieve real-time judgment, greatly reduce the huge loss caused by diseases and pests, which has important research value and significance. Based on the latest research results of detection theory CNN object detection and the characteristics of tomato diseases and pests images, this study will build the dataset of tomato diseases and pests under the real natural environment, optimize the feature layer of Yolo V3 model by using image pyramid to achieve multi-scale feature detection, improve the detection accuracy and speed of Yolo V3 model, and detect the location and category of diseases and pests of tomato accurately and quickly. Through the above research, the key technology of tomato pest image recognition in natural environment is broken through, which provides reference for intelligent recognition and engineering application of plant diseases and pests detection.
In this paper, image data of tomato diseases and insect pests under real natural environment were collected, and corresponding data processing was carried out to build tomato diseases and pests detection dataset. Based on the YOLO v3 model as the main body, the image pyramid structure is adopted to fuse features of different levels to obtain feature maps of different scales for location and category prediction. Then, the dimension of the object box is clustered, and the number of anchor box is increased, so that the model can obtain more edge information of the object. Finally, in the training process, multi-size images are used for training, so that the model can adapt to images of different resolutions. Experiments show that the improved YOLO v3 algorithm can improve the detection speed while ensuring the detection accuracy.
Related Work
Comparison between traditional machine learning technology and deep learning technology.
Before the development of deep learning technology, image recognition of plant diseases and insect pests was realized by traditional technology. With the development of deep learning technology, researchers begin to apply deep learning to the image recognition of diseases and pests, and have made a lot of achievements in recent years ( Mohanty et al., 2016 ; Sladojevic et al., 2016 ; Wang et al., 2017 ; Liu et al., 2018 ; Ferentinos, 2018 ; Brahimi et al., 2018 ; Kaur et al., 2019 ), especially in the disease and insect image recognition of apple, tomato, cucumber, and other common crops. The efficiency and effect of image recognition are much better than that of traditional recognition methods. The comparison between traditional recognition method and deep learning recognition method is shown in Table 1 .
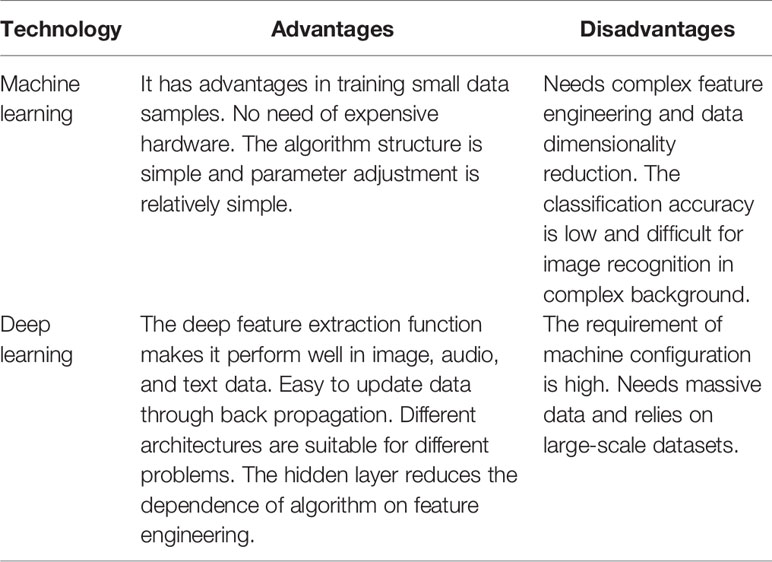
Table 1 Contrast between traditional machine learning and deep learning.
Object Detection Method of Plant Diseases and Insect Pests Based on CNN
Traditional machine vision methods have poor robustness in complex scenes, so it is difficult to meet the work requirements in complex scenes. The performance of CNN in image recognition has made great progress in the past few years, and in the ImageNet Large Scale Visual Recognition Challenge (ILSVRC), a lot of deep learning architectures have emerged, such as AlexNet ( Krizhenvshky et al., 2012 ), GoogleLeNet ( Szegedy et al., 2015 ), VGGNet ( Simonyan and Zisserman, 2014 ), ResNet ( Xie et al., 2017 ), and the accuracy of general image recognition is constantly being refreshed. CNN’s hard work and great breakthrough in the large-scale image classification competition prompted people to consider applying it to the problem of object detection. Different from image classification, object detection needs to detect and locate specific multiple objects from the image, which is mainly divided into two categories. One is to generate a series of candidate frames as samples by the algorithm, and then classify the samples by CNN, such as RCNN ( Girshick et al., 2014 ), faster RCNN ( Ren et al., 2016 ), and R-FCN ( Dai et al., 2016 ). The other one directly transforms the problem of object bounding box location into regression problem, which does not need to generate candidate boxes. The landmark algorithms include SSD ( Liu et al., 2016 ) and YOLO ( Redmon et al., 2016 ).
Arsenovic et al. (2019) established a plant disease dataset with 79,265 pieces collected under different meteorological conditions. A two-level structure, PlantDiseasenet, which is trained by PDNet-1 and PDNet-2 at the same time, is proposed. PDNet-1 uses the detection method proposed in the Yolo algorithm as the detection tool of plant leaves, and PDNet-2 is responsible for the classification of plant leaves. After training, the accuracy of the model is 93.67%. Jiang et al. (2019) proposed an improved CNN-based deep learning method for real-time detection of apple leaf diseases and insect pests. Firstly, through data expansion and image annotation technology, an apple leaf disease dataset (ALDD) composed of laboratory images and complex images under real field conditions is constructed. On this basis, a new method is proposed by introducing GoogleNet inception structure and rainbow concatenation. Finally, the proposed INAR-SSD (SSD with perception module and rainbow condition) model was trained to detect the five common apple leaf diseases and insect pests. The experimental results show that the model achieved 78.80% mAP and the detection speed is as high as 23.13 FPS. Tian et al. (2019) proposed a method of Apple Anthracnose damage detection based on deep learning and optimized the feature layer of Yolo V3 model with low resolution by using Densenet, which has greatly improved the utilization of neural network features and improved the detection results of Yolo V3 model. The experimental results show that the model achieved 95.57% mAP. Zheng et al. (2019) established a CropDeep species classification and detection dataset, including 31,147 images and more than 49,000 annotation examples from 31 different categories. These images are collected in real natural environment with different cameras, and the most advanced deep learning classification and detection model is used to provide a wide range of baseline experiments. The results show that the existing classification method based on deep learning can achieve more than 99% in classification accuracy, and only 92% in object detection accuracy. Meanwhile, Yolo V3 model has good application potential in agricultural detection tasks.
Object Detection of Tomato Diseases and Insect Pests Based on CNN
Fuentes et al. (2017) proposed a real-time system for the identification and location of tomato plant diseases and insect pests. The system is consisted of three network structures, Fast R-CNN, SSD, and R-FCN are compared. Each network structure is combined with different feature extraction networks (such as VGG, RESNET). The system has the following advantages: (1) the images used in the system are collected in the field; (2) considering that a crop may be affected by multiple diseases and insect pests at the same time; (3) the system has strong robustness and can be used in the actual field environment to achieve detection; (4) the system can also solve complex tasks, such as infection status (early, late), infection location (leaves, culm, fruit) and both sides of leaves (obverse and reverse). Fuentes et al. (2018) proposed an improved framework of tomato plant diseases and insect pests detection algorithm aiming at the problem of false alarm and unbalanced classification, which is composed of three main units: (1) the main diagnosis unit (bounding box generator), which generates the bounding box containing the location of infected area and class; (2) the auxiliary diagnosis unit (CNN filter bank), which trains each independent CNN to filter the samples of misclassification; (3) the integration unit, which combines the information of the autonomous diagnosis unit and the auxiliary diagnosis unit, and keeps the true positive samples at the same time, so as to eliminate the false positive of the classification errors in the first unit. The experiment shows that the recognition rate of this method is about 96%. Fuentes et al. (2019) proposed a method which can not only effectively detect and locate plant anomalies, but also generate diagnostic results, display the location of anomalies and describe the symptoms of sentences as output, and has achieved 92.5% average precision (mAP) in the newly created tomato plant anomaly description dataset. However, it uses Faster R-CNN to detect the object, which needs to be carried out in two steps. First, extract the region proposal, and then detect it. However, Yolo directly generates coordinates and probability of each category through expression once. Therefore, the real-time performance of existing research needs to be improved.
Problems of Existing Research and Development Trends
The development of the existing research provides a reference and feasibility basis for the application of CNN in the detection of plant diseases and pests, and can avoid the shortcomings of the traditional machine vision method in the feature extraction process. Through CNN object detection technology, it can greatly facilitate plant breeding and help farmers to supervise their fields better. However, the automatic identification and detection of plant diseases and insect pests are mainly faced with the following difficulties ( Barbedo, 2016a ; Barbedo, 2016b ; Barbedo, 2017 ; Barbedo, 2018a ; Barbedo, 2018b ).
i. The background of the image is complex. In addition to the infected leaves, the image may contain other elements, such as crop stalks, soil, etc. Especially for the photos taken in the field environment, not only the background is messy, there are also factors such as light, angle difference, etc.
ii. There may be no clear boundary between the infected area and the healthy area.
iii. The same disease has different characteristics in different stages of development, even in different locations.
iv. The characteristics of different kinds of diseases and insect pests may be the same or slightly different, and there may be multiple diseases and insect pests at the same location at the same time.
v. It is difficult to distinguish the infected area from other dead plant tissues.
vi. Throughout the different stages of development of the insect (nymph or larva and adult) and the different instars, the morphological characteristics of insect vary greatly. Especially for small target pests, it is difficult to identify.
At present, the research of plant diseases and insect pests based on deep learning involves a wide range of crops, including all kinds of vegetables, fruits, and food crops. The completed tasks not only include the basic tasks of classification and detection of diseases and insect pests, but also more complex tasks such as the judgment of infection degree. Because deep learning relies on large-scale datasets, there are few open datasets of plant diseases and insect pests at present, the researchers usually find the best solution by comparing different training set and test set proportion and using different network models. However, there is still a certain gap between the complexity of these susceptible images and the real field scene, and there is a certain gap between the real-time detection of diseases and pests in fields based on mobile devices.
The Principle Of Yolo V3 Model
Yolo v3 design idea.
Yolo algorithm proposed by Redmon et al. (2016) in 2016, the object detection task is transformed into regression problem, which greatly speeds up the detection speed. Yolo V3 ( Redmon and Farhadi, 2018 ) was proposed on the basis of Yolo V2 ( Redmon and Farhadi, 2016 ), the detection speed of Yolo V2 is maintained, and the detection accuracy is greatly improved. Yolo V3 uses the idea of residual neural network ( He K et al., 2016 ). The introduction of multiple residual network modules and the use of multi-scale prediction improve the shortcomings of Yolo V2 network in small object recognition. Because of the high accuracy and timeliness of detection, this algorithm is one of the best algorithms in the field of object detection. This model uses a lot of 3 × 3 and 1 × 1 convolution layers with good performance, and some residual network structures are also used in the subsequent multi-scale prediction. Finally, it has 53 convolution layers, so it can also be called Darknet-53.
Yolo V3 network introduces the idea of using anchor boxes in Faster R-CNN. For coco dataset and VOC dataset, three scales are used for prediction. Each scale has three anchor boxes, and the feature map with large scale uses small priori box, so you can select the appropriate priori box according to the target you want to identify, and the network structure can be changed according to the scale that needs to predict.
Feature Extraction Network Darknet-53
Yolo V3 adopts the network structure of Darknet-53, which consists of 53 (2 + 1*2 + 1 + 2*2 + 1 + 8*2 + 1 + 8*2 + 1 + 4*2 + 1 = 53) convolution layers. The network structure contains 53 convolutional layers and 5 maximum pooling layers. Batch normalization and dropout operations are added after each convolutional layer to prevent overfitting. Darknet-53 is composed of five residual blocks, which uses the idea of residual neural network for reference. Figure 1 shows the Darknet-53 network structure. Yolo V3 has increased the network depth by introducing residual unit to avoid gradient disappearance.
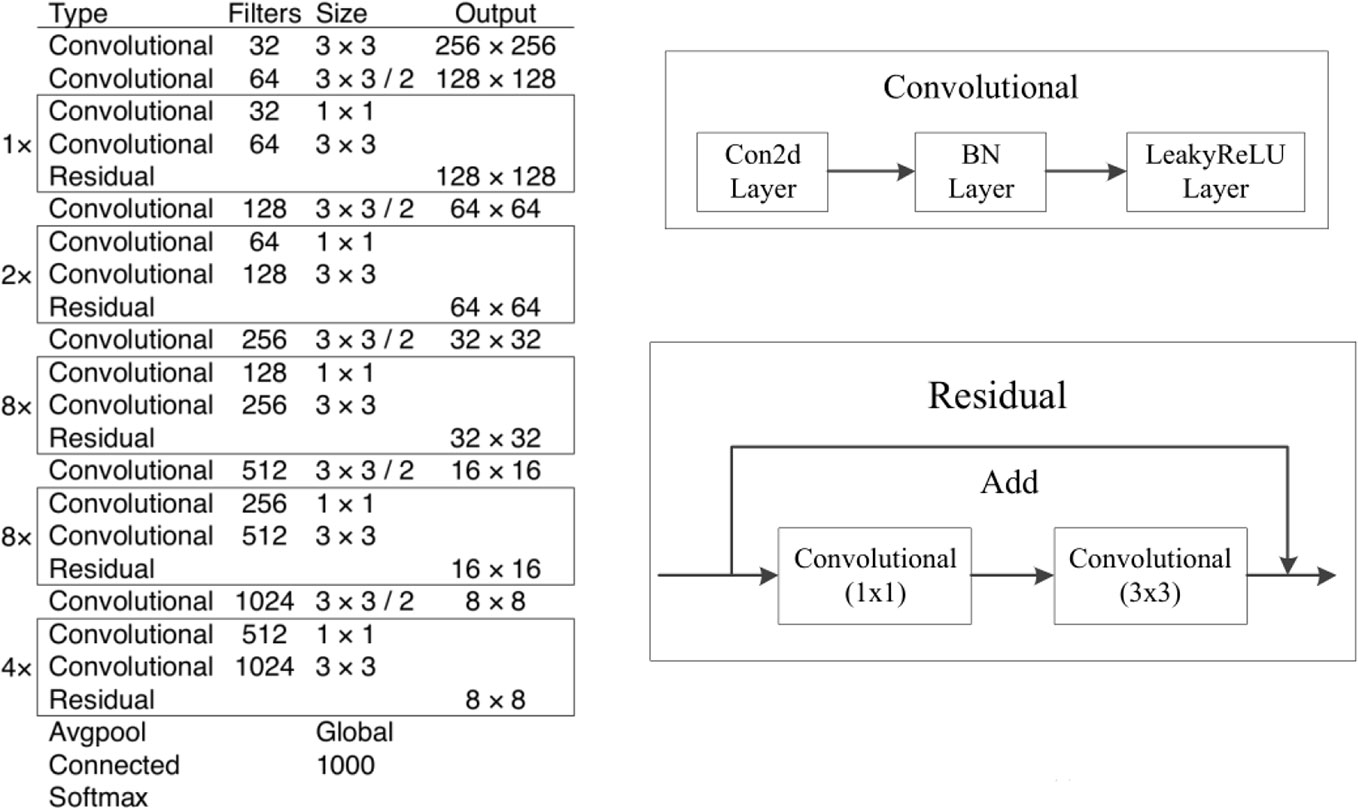
Figure 1 Darknet-53 network structure.
The Method Of Improving Yolo V3 Model
Compared with the whole tomato plant image, the disease spots and pests are small objects. Therefore, Yolo V3 model is a little inadequate in the scale when recognizing tomato disease spots and pests. In view of this situation, Yolo V3 model is improved to adapt to specific tomato diseases and pests detection tasks. The improved Yolo V3 network structure is shown in Figure 2 .
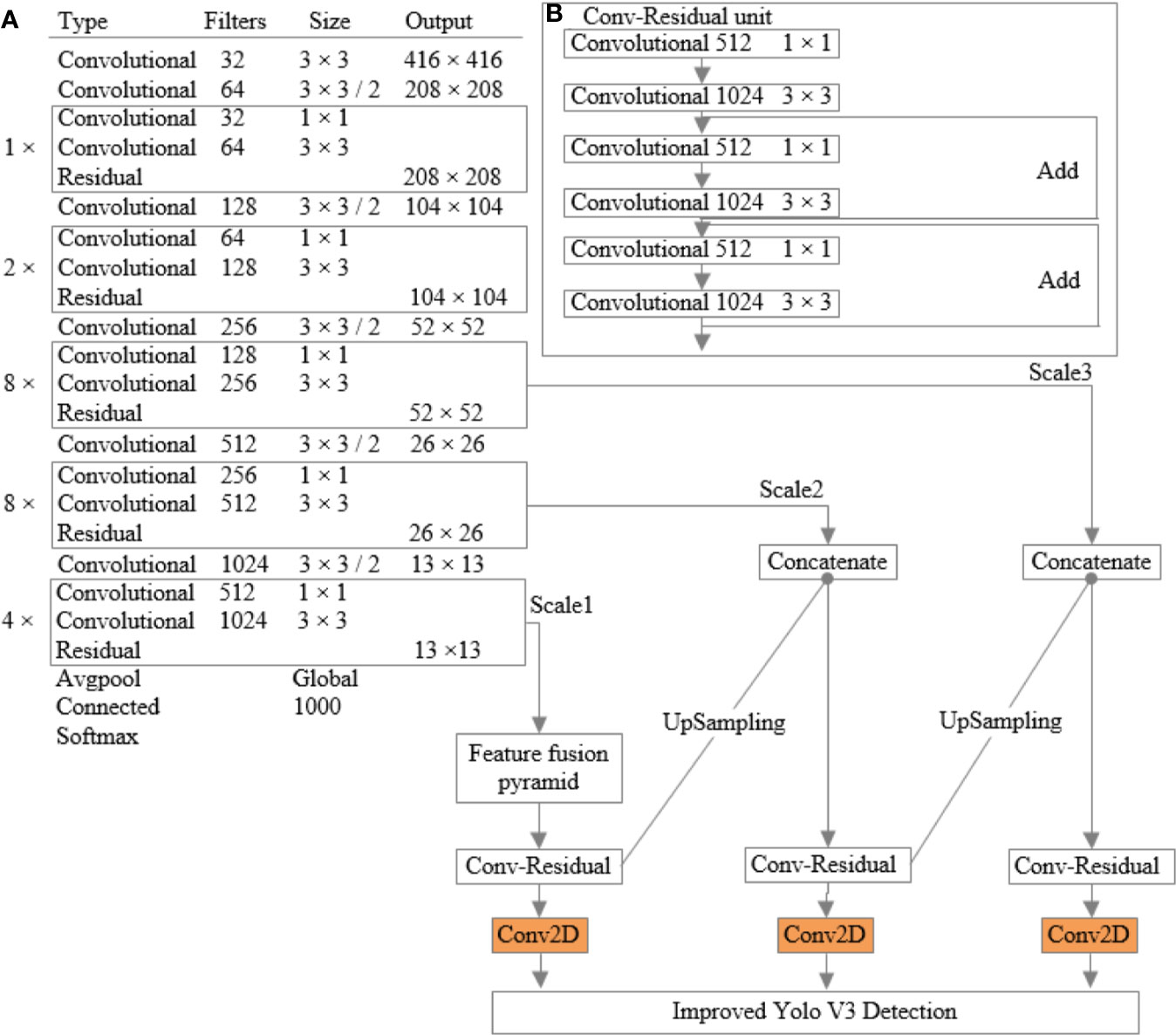
Figure 2 The improved Yolo V3 network structure (A) network structure; (B) conv-residual unit.
The improved Yolo V3 network structure uses feature fusion to increase the number of feature maps, which saves a lot of computation and is very important for improving the running speed. Two residual units were added to the detection network to further enhance the expression of image features. Also, the image pyramid structure and improved conv-residual unit are used to reduce the impact of depth reduction on the precision of the backbone network. Therefore, the improved Yolo V3 network structure inherits Darknet-53’s structure in ideology but reduces the dependence of the whole network on ResNet, and optimize the network forward propagation speed and model size, making it more suitable for mobile platforms with weak computing power.
Based on the image pyramid structure, Yolo V3 model is improved. Upper sampling is used to fuse the high-level features with the low-level features, and three sets of prediction feature maps of different scales are obtained. Then, the location and category are predicted on these three sets of prediction feature maps.
K-means algorithm is used to calculate the prior box dimension in the self-built tomato diseases and pests dataset. The priori box dimension obtained in Yolo V3 model is trained on the COCO dataset, and its parameters are divided into three different scales. For the specific task of tomato diseases and pests detection, cluster operation should be carried out for the specific dataset, and smaller priori box should be used for the larger scale feature map to get the corresponding cluster center.
The multi-scale training strategy was used to train the self-built dataset of tomato diseases and insect pests. In Yolo V3 model, the full connection layer is removed and uses the full convolution operation. In the training process, the input size can be changed at any time, so that the trained model can adapt to different scales of tomato diseases and pests images.
Multiscale Feature Detection Based on Image Pyramid
In Yolo V3, Darknet-53 network is used to extract features, and feature visualization technology is used to clearly show the effect of each level of features. Low-level features have rich details and location information, while high-level features have rich semantic features. From low-level to high-level, the details are decreasing, while the semantic information is increasing. For location prediction, more low-level feature information is needed, for category prediction, more high-level local information is needed. Therefore, based on the image pyramid model, the up sampling method is used to fuse the high-level features with the low-level features to obtain the feature maps of different scales for location and category prediction.
The feature pyramid on the right side of Figure 3 is generated by the feature pyramid on the left side. The whole process is as follows: first, the input image is deeply convoluted, then the features on layer2 are convoluted, and the features on layer4 are sampled to make them have the same size. Then, the processed layers layer2 and layer4 are convoluted and operated to input the obtained results to layer5. In the same way, feature fusion is carried out among multiple layers to get multiple sets of feature maps for prediction. Based on this scheme, the processed low-level features and high-level features are accumulated. The purpose of doing this is that because the low-level features can provide more accurate location information, and multiple down sampling and up sampling operations make the location information of the deep-seated network have errors. Therefore, they are combined to build the deep feature pyramid, and integrate the multi-layer feature information on different feature maps for category and location prediction.
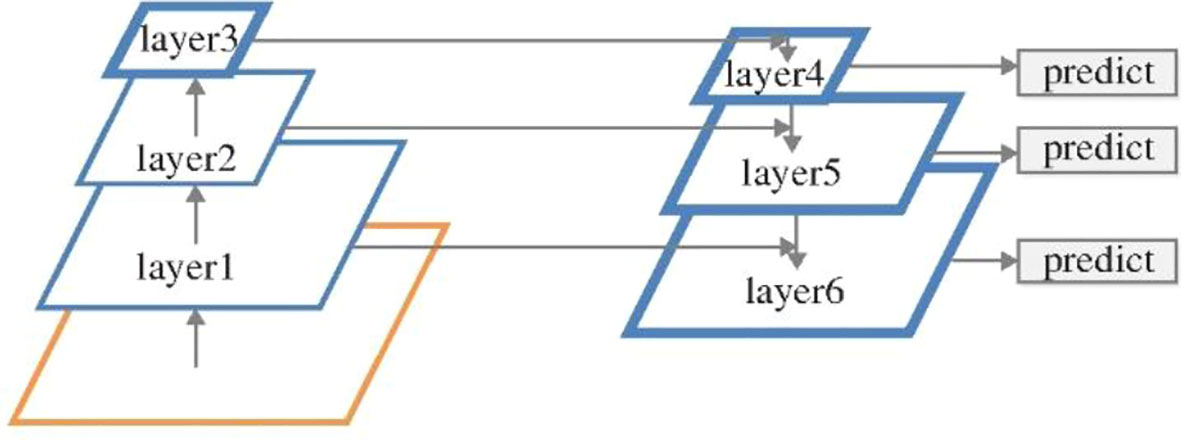
Figure 3 Feature Fusion Pyramid.
Based on the above idea of feature fusion, Yolo V3 algorithm is improved. Up sampling is used to fuse high-level features with low-level features. Finally, three sets of feature maps are obtained, and these three sets of feature maps of different scales are used for prediction. The improved network structure is shown in Figure 4 .
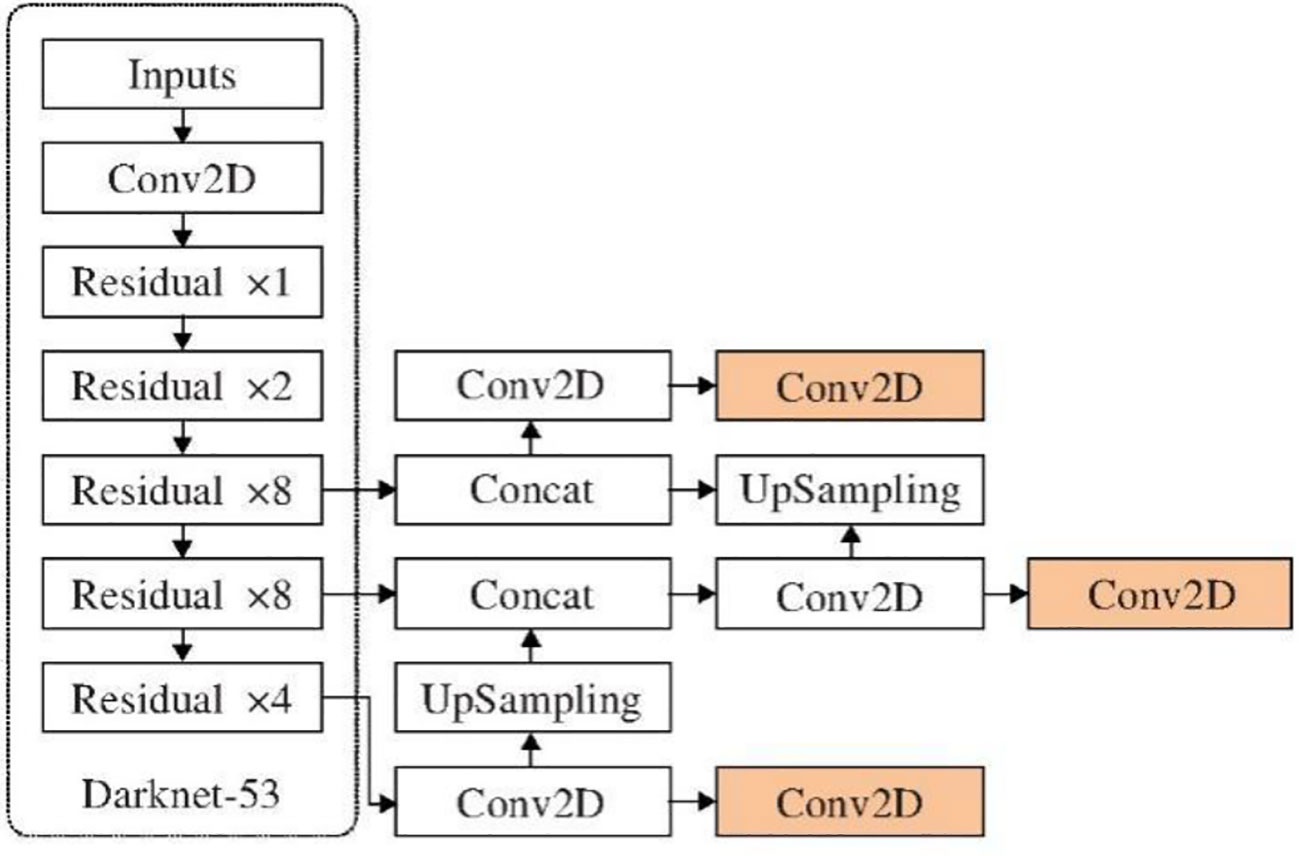
Figure 4 Improved network structure.
Specific network structure improvement details: first, get the characteristic pyramid through the Darknet-53, and carry out continuous 1 × 1 and 3 × 3 convolution operations on the conv53 layer to get a group of Yolo layers to be processed, and then carry out a group of 1 × 1 and 3 × 3 convolution operations on the layer to get a small scale Yolo layer. At the same time, perform upper sampling operation on this layer, and carry out convolution operation with the conv45 layer in the Darknet-53, as well using continuous 1 × 1 and 3 × 3 convolution operations, the second group of Yolo layers to be processed are obtained, and a group of 1 × 1 and 3 × 3 convolution operations are performed on this layer to obtain the mesoscale Yolo layer. At the same time, perform upper sampling operation on this layer, convoluted and operated with conv29 layer in Darknet-53, and the third group of Yolo layers to be processed are also obtained by using continuous 1 × 1 and 3 × 3 convolution operations, and a group of 1 × 1 and 3 × 1 layers are performed on this layer 3 convolution operation to get large scale Yolo layer. After the above operations, three sets of Yolo feature layers with different scales are obtained, and these three sets of feature layers are used for location and category prediction.
K-Means Dimension Clustering Algorithm
Yolo V3 uses the prior box to predict the coordinates of the bounding box in Yolo V2. The difference is that Yolo V3 uses k-means algorithm to predict and gets nine prior boxes, and divides them into three scale feature maps, among which the larger scale feature map uses the smaller prior box to obtain more edge information of the object.
The dimensions of nine sets of prior boxes calculated in Yolo V3 are respectively as follows: (10, 13), (16, 30), (33, 23), (30, 61), (62, 45), (59, 119), (116, 90), (156, 198), and (373, 326). However, in the actual detection task of tomato diseases and insect pests, the prior box dimension calculated by Yolo V3 algorithm is not suitable for the detection scene of tomato diseases and insect pests, so it is difficult to get accurate object bounding box information by using the original prior box dimension in Yolo V3 algorithm.
Therefore, in the tomato diseases and pests detection scenario, K-means algorithm is used to cluster the self-built tomato diseases and pests dataset, and nine sets of prior box dimension centers are obtained respectively as follows: (44, 11), (53, 18), (60, 22), (73, 25), (78, 27), (81, 31), (89, 41), (97, 43), and (109,51). The region is arranged from small to large, and divided into feature maps of three different scales, among which the larger scale feature map uses the smaller prior frame. Finally, the cluster center is used to detect tomato diseases and insect pests.
Multiscale Training
In the Yolo detection algorithm, convolutional network is used to extract the features, and then the full connection layer is used to get the prediction value. However, due to the existence of full connection layer, the input image size of the network must be fixed in the training process, so the final training network does not have robustness to different sizes of test images.
According to the self-built tomato diseases and pests dataset, the size of the input image is different. Therefore, in order to enhance the robustness of the model to different image sizes, multi-scale training strategy is adopted. Specifically, remove the full connection layer in Yolo V3 network and change it to full convolution operation. Figure 5 shows the process of converting a full connection layer to a convolution layer.
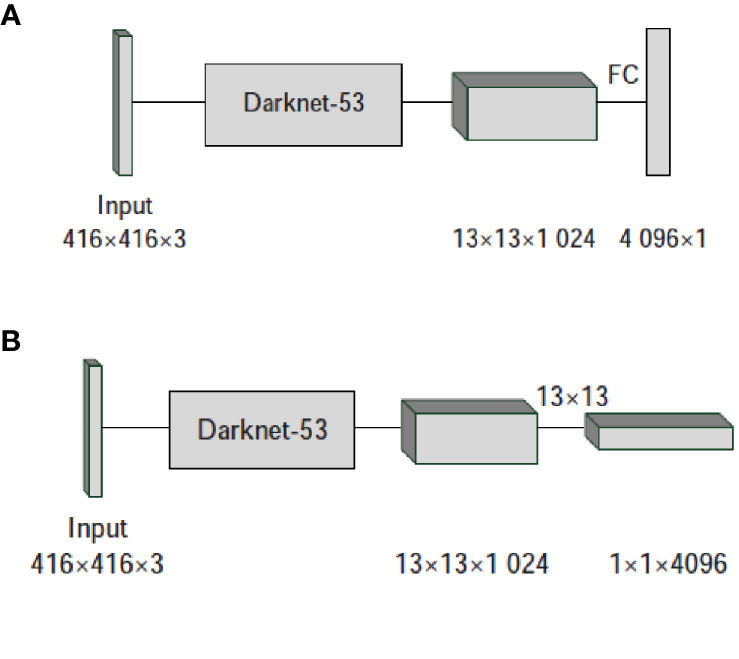
Figure 5 Conversion of full connection layer to convolution layer. (A) Full connection operation. (B) Convulation operation.
Figure 5A uses the full connection layer for prediction, and Figure 5B converts the full connection layer into a convolution layer for prediction. When the input image size is 416 × 416, after passing through the Darknet-53 network, 13 × 13 × 1024 feature maps are output. Passing through a full connection layer containing 4,096 neurons, a set of 4,096 × 1 feature maps are obtained in Figure 5A . Figure 5B uses 4,096 convolution kernels of 13 × 13, and finally obtains 1 × 1 × 4,096 feature maps, which is essentially equivalent to 4,096 nerves obtained by full connection. For the above two network structures, when the image input size is 416 × 416, the network can operate normally, but there are other sizes of pictures in the self-built tomato diseases and pests dataset. For example, when inputting 608 × 608 size images, after passing through the Darknet-53 network, the 19 × 19 × 1024 feature map is output. For the structure of Figure 5A , the next step is to connect the feature map with 4,096 neurons. The size of the original architecture is 13 × 13, and now it is 19 × 19. Therefore, during the propagation of the network, the previous parameter matrix cannot be used, and the network cannot operate normally. For the structure of Figure 5B , after the full connection is changed to convolution operation, the network can continue to run, and finally get the correct output of 7 × 7 × 4,096. Therefore, after the full connection layer is changed to full convolution operation, input images of different sizes are used for training, and the improved algorithm can adapt to different sizes of test images.
In addition, after changing the full connection layer to full convolution operation, the performance of the network will be improved. When using the full connection layer in Figure 5A in the forward propagation operation of the network, it can be calculated that 708,837,377 parameters are needed. While using the full convolution operation in Figure 5B , only 696,320 parameters are needed. Therefore, after converting the full connection layer into convolution operation, the number of parameters can be reduced, the network operation amount can be reduced, and the network performance can be improved.
Based on the above analysis, this paper will use multi-scale training strategy to train the self-built tomato diseases and pests dataset. Because the whole network has five maximum pool layers, the down sampling rate of the network is 32. In the training process, the input size of the training picture of the dataset of tomato diseases and pests is divided into a series of values of multiple of 32, and the calculation formula of the size is as follows:
Among them, S n is the size of the group n input image. During network initialization, S 1 is 320 × 320. Through formula (1), it can be concluded that the size of the input image is: 320, 352, 384, 416, 448, 480, 512, 544, 576, and 608. In the process of training, one kind of input image size is selected randomly every 10 rounds to achieve the effect that the model can adapt to images of different sizes.
Experimental Results And Comparative Analysis
Experimental operation environment.
The experimental hardware environment of this paper is shown in Table 2 . On this basis, the software environment is built as follows: Ubuntu 16.04, python, OPPENCV, CUDA, etc. The framework uses the Caffe framework and Darknet-53 framework.
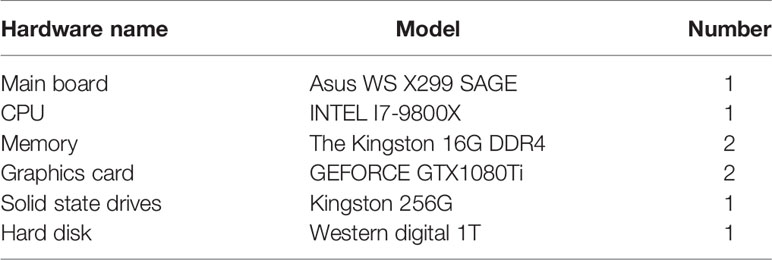
Table 2 Configuration of experimental hardware environment.
Experimental Dataset Building
In the process of building tomato diseases and pests detection dataset, different equipment including monitoring, digital camera, and smart phone are used to collect tomato plant photos in the local tomato planting greenhouses. In different time periods, different weather conditions and different scenes, single frame images and photos in the monitoring video are randomly selected as the dataset. The number of samples of each category is shown in Table 3 . The labeling tool is used to mark the images. With this tool, when the manual operation is carried out, we only need to mark the user-defined objects in the image, and the tool can automatically generate the corresponding configuration file.
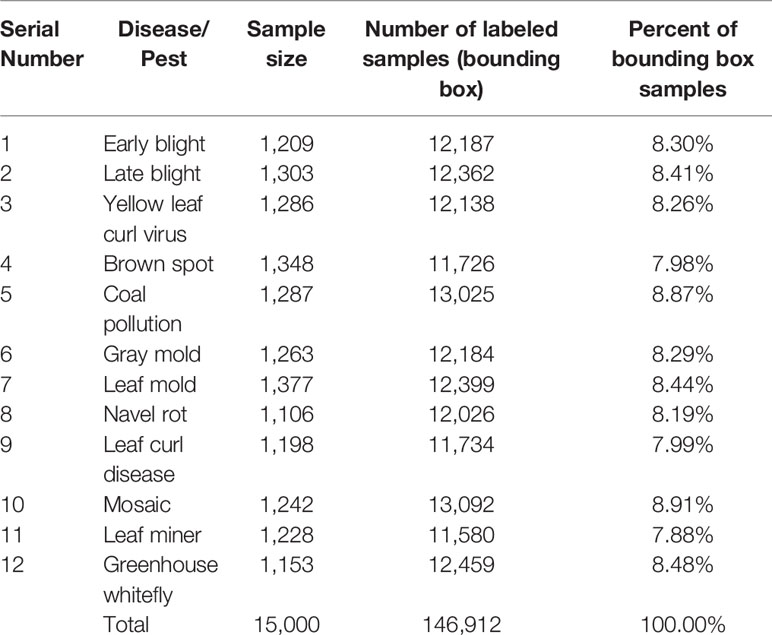
Table 3 Number of samples of each disease type.
Model Training
Use the weight parameters provided on Yolo V3 official website as the initialization parameters of network training, randomly use the images in the self-built tomato diseases and pests detection training dataset to finetune the network parameters, so that the detection effect of the whole model is optimal, and the model parameter settings are shown in Table 4 .
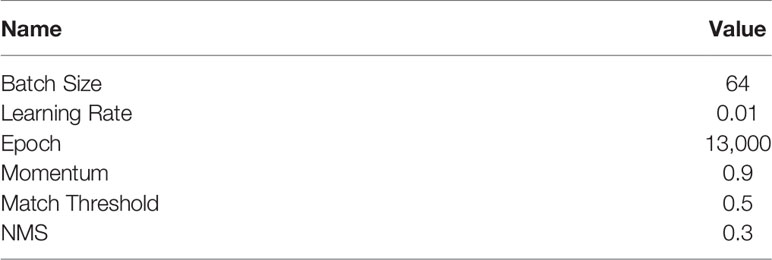
Table 4 Model parameter settings.
Comparative Analysis of Experiments
In this paper, Yolo V3 algorithm is mainly used for the experiment. The improvements include multi-scale feature detection based on image pyramid, object frame dimension clustering, and multi-scale training. At the same time, in order to verify the validity, accuracy and stability of the model, SSD, Faster R-CNN, and Yolo V3 algorithms are used for experimental comparative analysis, and the detection accuracy mAP (Mean Average Precision) and detection time are used as the evaluation indexes of detection effect. The results are shown in Table 5 .
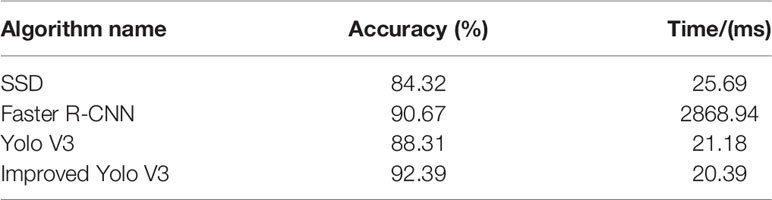
Table 5 Comparison of experimental results.
It can be seen from the experimental results that in terms of object detection accuracy, Faster R-CNN and the improved Yolo V3 are superior to the other two algorithms. However, due to the need to establish RPN network in the process of object detection, which involves a lot of calculation, Faster R-CNN is inferior to the improved Yolo V3 in detection speed. In general, the improved Yolo V3 algorithm is superior to the other three algorithms in detection accuracy and speed, especially the detection time is the shortest. Therefore, the improved Yolo V3 performs best for real-time detection tasks. The improved Yolo V3 algorithm can complete the detection task of tomato diseases and insect pests well on the premise of considering both the detection accuracy and detection speed.
The loss curves of the four algorithms are shown in Figure 6 . It can be seen that the loss of the algorithm in this paper is the smallest, while Faster R-CNN is the largest. Therefore, the convergence speed of this algorithm is the fastest.
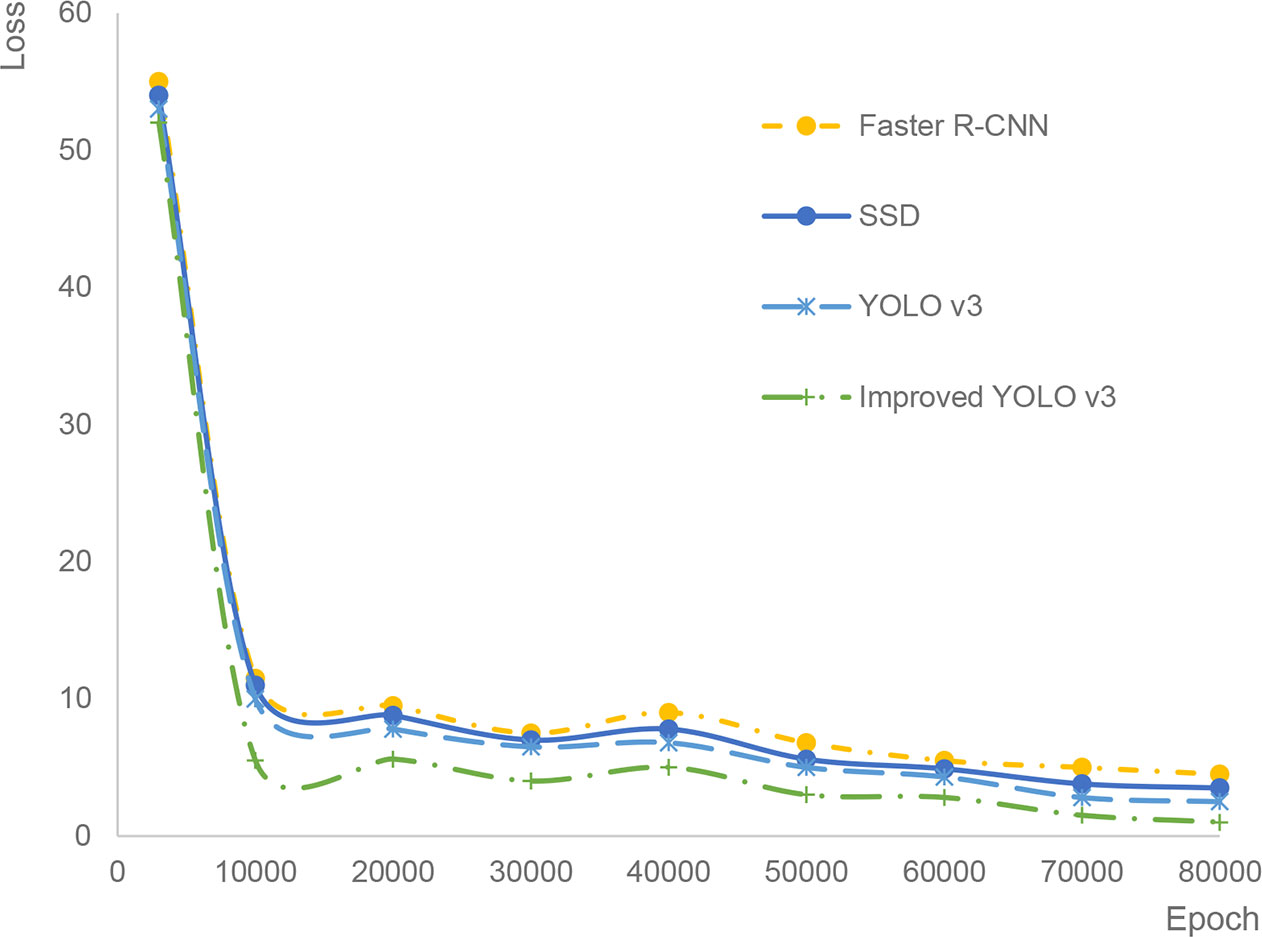
Figure 6 Loss function contrast.
Performance Analysis in Small Object Scenario
Performance analysis in small object scenario is important for different sizes of diseases and pests objects throughout their different stages. The multi-scale detection method based on image pyramid proposed in this paper improves the detection effect of small objects. In order to verify the detection effect of the small object detection performance, the test dataset is sorted according to the size of the object. The 0-10%, 10% - 30%, 30% - 70%, 70% - 90% of the object size are divided into five sub categories: XS, S, M, L, XL, which represent the size of different objects. Figure 7 shows the detection performance of the original Yolo V3 algorithm and the improved algorithm for objects of different sizes.
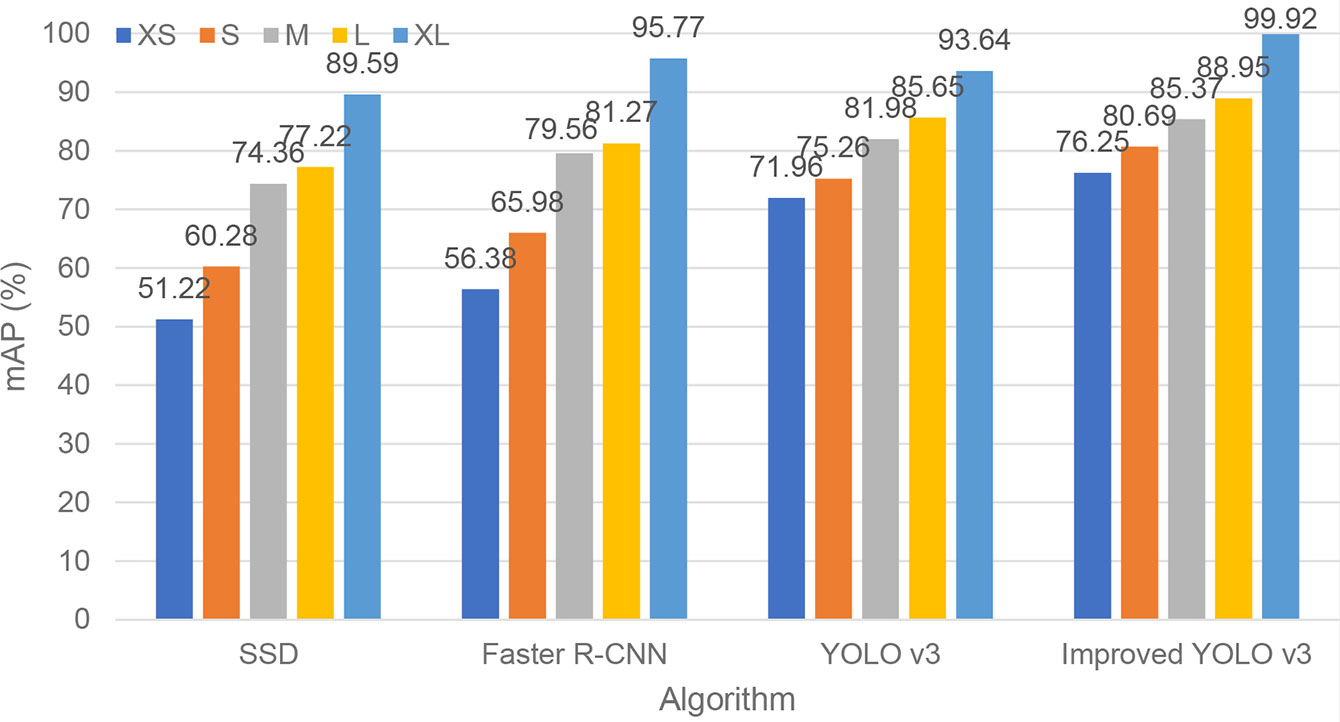
Figure 7 Object size sensitivity analysis of four algorithms.
As can be seen from Figure 7 , the detection accuracy of the improved Yolo V3 algorithm for objects of different sizes is higher than that of the other three algorithms. Therefore, when detecting tomato diseases and insect pests, using the strategy of multiscale feature fusion, combining the high-level features with the low-level features, the improved algorithm has achieved the best results in the detection of small objects. Throughout the different stages of development of the insect (nymph or larva and adult) and the different instars, the morphological characteristics of insect vary greatly. For small object pests, the improved algorithm can achieve good results.
Performance Analysis of Different Resolution Images
The multi-scale training method proposed in this paper can enhance the robustness of the model for the detection of different resolution images. In order to verify the detection effect of the model on the input images of different resolutions, this paper divides the test dataset images into three different resolution sizes, namely {320, 608, 1024}, representing three types of images: low resolution, medium resolution and high resolution. Figure 8 represents the detection accuracy performance of four algorithms for different resolution images.
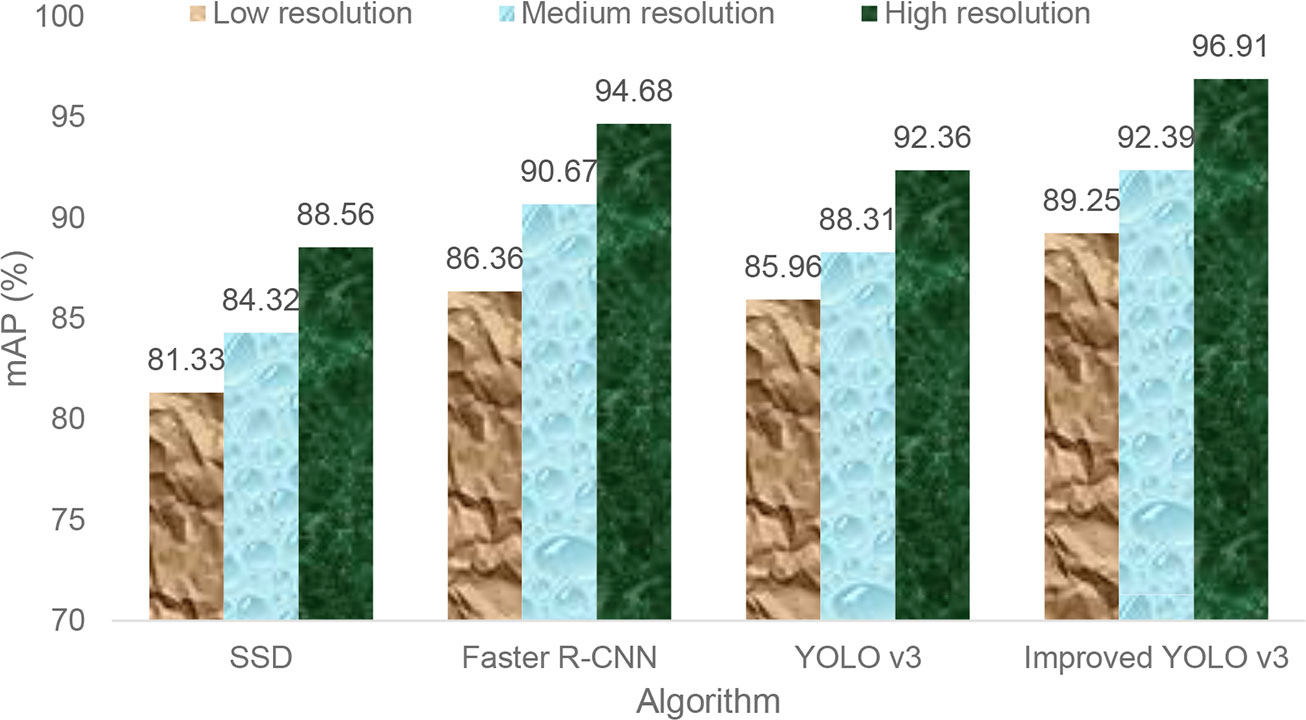
Figure 8 Object image resolution sensitivity analysis of four algorithms.
As can be seen from Figure 8 , the detection accuracy of the improved Yolo V3 algorithm for different resolution images is higher than that of the other three algorithms. It can be seen that the multi-scale training detection strategy adopted in this paper not only can enhance the adaptability of the model to different resolution images, but also can detect the location and category of tomato diseases and insect pests accurately and quickly.
In addition, in order to show the detection effect of the algorithm in this paper more intuitively, some detection images are selected, as shown in Figure 9 . It can be seen that the algorithm in this paper can correctly detect the location and category of the object when there are multiple objects and small objects in the image, and can effectively avoid the problem of false detection and missing detection.
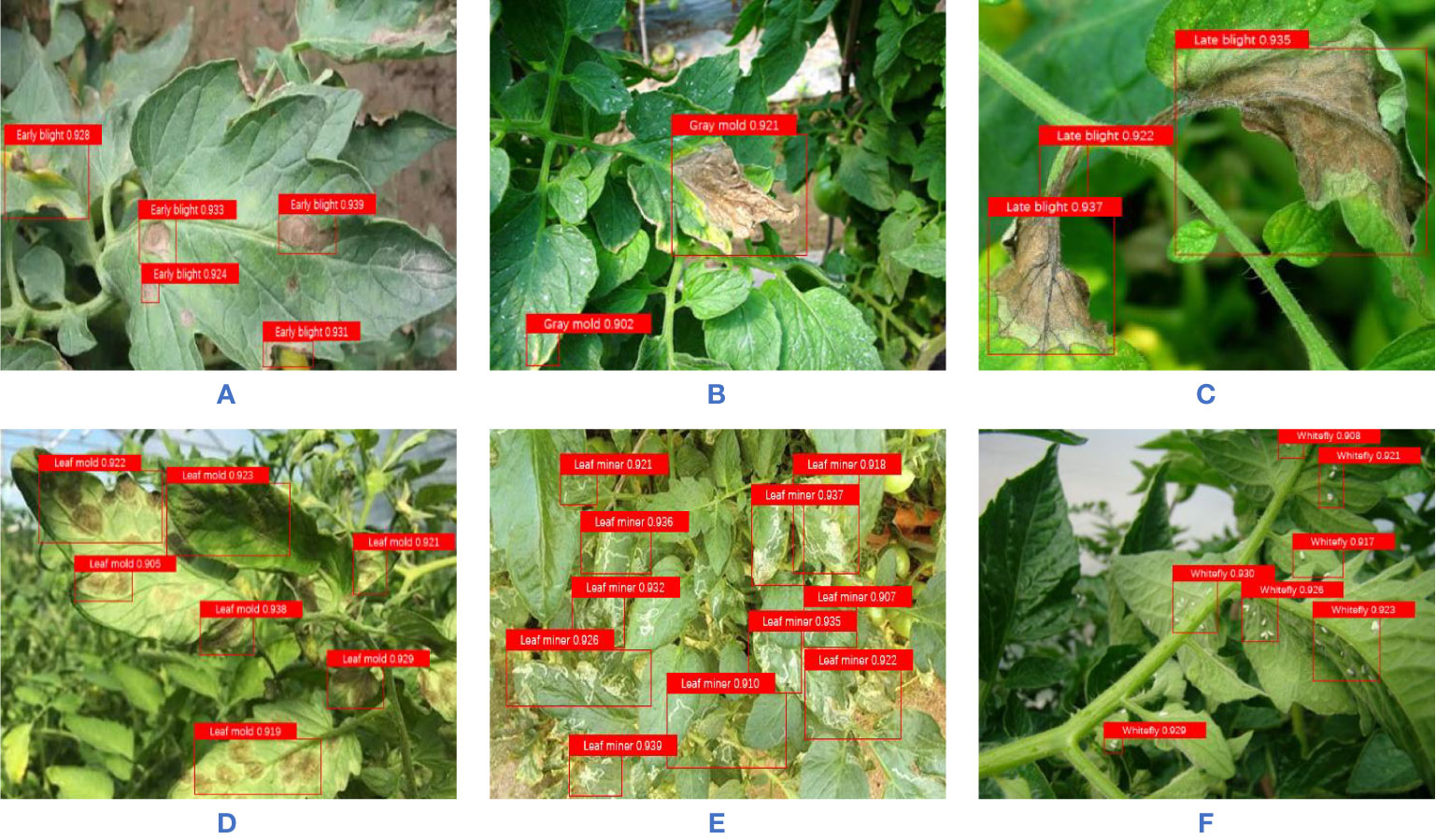
Figure 9 The detection effect diagram of the improved YOLO v3 algorithm (A) Early bight; (B) Gray mold; (C) Late blight; (D) Leaf mold; (E) Leaf miner; (F) Whitefly.
Conclusions and Future Directions
Conclusions.
1. In this paper, an improved Yolo V3 algorithm is proposed to detect tomato diseases and insect pests. Yolo V3 network was improved by using multi-scale feature detection based on image pyramid, object bounding box dimension clustering and multi-scale training. The experimental results show that the detection accuracy of the algorithm is 92.39% and the detection time is only 20.39 ms. Therefore, for the task of tomato diseases and pests detection, the improved Yolo V3 algorithm proposed in this paper can not only maintain a high detection rate, but also meet the real-time detection requirements, and can detect the location and category of tomato diseases and insect pests accurately and quickly.
2. Compared with SSD, Faster R-CNN and the original Yolo V3, the improved Yolo V3 CNN can achieve higher detection accuracy and shorter detection time, and meet the requirements of real-time detection accuracy and speed of tomato diseases and pests.
3. Performance analysis in small object scenario and different resolution images further verifies that the improved Yolo V3 network has strong robustness for detection of different object sizes and different resolution images in complex environment, and has high detection and positioning accuracy, which can meet the needs of tomato diseases and pests detection in complex environment.
Future Directions
1. In different growth cycles, the appearances of diseases and pests are different. Therefore, the images of diseases and pests should be divided more carefully, and the same class of diseases and pests should be divided according to the growth period as the standard. In the future, the division of dataset will be improved.
2. In order to make the model more widely applied, the next work will collect a large number of high-quality images of different types of diseases and pests, and proceed to insert other insect pests, optimize and adjust the model, and extend this to other crops, so as to improve the practicability and accuracy of crop diseases and pests image recognition.
3. Construct an intelligent patrol robot for tomato diseases. In a real greenhouse tomato planting base, the intelligent patrol inspection robot for Tomato Diseases can work around the clock. The utility of the improved Yolo V3 model will be further enhanced by carrying a mobile robot arm with a detection sensor for disease detection. The purpose of early detection and early diagnosis of tomato diseases will be achieved by capturing the lesions in real time and non-destructive detection of tomato diseases.
Data Availability Statement
The datasets analyzed in this article are not publicly available. Requests to access the datasets should be directed to [email protected] .
Author Contributions
JL designed research. JL and XW conducted experiments and data analysis and wrote the manuscript. XW revised the manuscript. All authors contributed to the article and approved the submitted version.
This study is supported by the Facility Horticulture Laboratory of Universities in Shandong with project numbers 2019YY003, 2018RC002, 2018YY016, 2018YY043, and 2018YY044; Research and Development Plan of Applied Technology in Shouguang with project number 2018JH12; 2018 innovation fund of science and technology development center of the China ministry of education with project number 2018A02013; 2019 basic capacity construction project of private colleges and universities in Shandong province; Weifang Science and Technology Development Program with project numbers 2019GX081 and 2019GX082. Key research and development plan of Shandong Province with project number 2019RKA07012 and 2019GNC106034.
Conflict of Interest
The authors declare that the research was conducted in the absence of any commercial or financial relationships that could be construed as a potential conflict of interest.
Arsenovic, M., Karanovic, M., Sladojevic, S., Anderla, A., Stefanović, D. (2019). Solving Current Limitations of Deep Learning Based Approaches for Plant Disease Detection. Symmetry 11, 21. doi: 10.3390/sym11070939
CrossRef Full Text | Google Scholar
Barbedo, J. G. A. (2016a). A novel algorithm for semi-automatic segmentation of plant leaf disease symptoms using digital image processing. Trop. Plant Pathol. 41 (4), 210–224. doi: 10.1007/s40858-016-0090-8
Barbedo, J. G. A. (2016b). A review on the main challenges in automatic plant disease identification based on visible range images. Biosyst. Eng. 144, 52–60. doi: 10.1016/j.biosystemseng.2016.01.017
Barbedo, J. G. A. (2017). A new automatic method for disease symptom segmentation in digital photographs of plant leaves. Eur. J. Plant Pathol. 147 (2), 349–364. doi: 10.1007/s10658-016-1007-6
Barbedo, J. G. A. (2018a). Factors influencing the use of deep learning for plant disease recognition. Biosyst. Eng. 172, 84–91. doi: 10.1016/j.biosystemseng.2018.05.013
Barbedo, J. G. A. (2018b). Impact of dataset size and variety on the effectiveness of deep learning and transfer learning for plant disease classification. Comput. Electron. Agric. 153, 46–53. doi: 10.1016/j.compag.2018.08.013
Brahimi, M., Arsenovic, M., Laraba, S., Sladojevic, S., Boukhalfa, K., Moussaoui, A. (2018). “Deep learning for plant diseases: detection and saliency map visualisation,” in Human and Machine Learning. Eds. Zhou, J., Chen, F. (Cham, Switzerland: Springer International Publishing), 93–117.
Google Scholar
Dai, J., Li, Y., He, K., Sun, J. (2016). R-FCN: Object Detection via Region-based Fully Convolutional Networks. arXiv. arXiv:1605.06409v2.
Diaz-Pendon, J. A., Canizares, M. C., Moriones, E., Bejarano, E. R., Czosnek, H., Navas-Castillo, J. (2010). Tomato yellow leaf curl viruses: Menage a trois between the virus complex, the plant and whitefly vector. Mol. Plant Pathol. 11, 414–450. doi: 10.1111/j.1364-3703.2010.00618.x
Ferentinos, K. P. (2018). Deep learning models for plant disease detection and diagnosis. Comput. Electron. Agric. 145, 311–318. doi: 10.1016/j.compag.2018.01.009
Food and Agriculture Organization of the United Nations. Plant Diseases and pests. Available online: http://www.fao.org/emergencies/emergency-types/plant-pests-and-diseases/en/ (accessed on 5 February 2020).
Fuentes, A., Yoon, S., Kim, S. C., Park, D. S. A. (2017). Robust Deep-Learning-Based Detector for Real-Time Tomato Plant Diseases and Pests Recognition. Sensors 17, 2022. doi: 10.3390/s17092022
Fuentes, A. F., Yoon, S., Lee, J., Park, D. S. (2018). High-performance deep neural network-based tomato plant diseases and pests diagnosis system with refinement filter bank. Front. Plant Sci. 9, 1162. doi: 10.3389/fpls.2018.01162
PubMed Abstract | CrossRef Full Text | Google Scholar
Fuentes, A. F., Yoon, S., Park, D. S. (2019). Deep Learning-Based Phenotyping System With Glocal Description of Plant Anomalies and Symptoms. Front. Plant Sci. 10, 1321. doi: 10.3389/fpls.2019.01321
Gilbertson, R. L., Batuman, O. (2013). Emerging Viral and Other Diseases of Processing Tomatoes: Biology Diagnosis and Management. Acta Hortic. 1, 35–48. doi: 10.17660/ActaHortic.2013.971.2
Girshick, R., Donahue, J., Darrell, T., Malik, J. (2014). Rich feature hierarchies for accurate object detection and semantic segmentation. arXiv , 580–587.
He, K. M., Zhang, X. Y., Ren, S. Q., Sun, J. (2016). Deep residual learning for image recognition [C]//2016 IEEE Conference on Computer Vision and Pattern Recognition (CVPR), Las Vegas, NV, USA. New York: IEEE, June 27–30, 2016. pp. 770–778.
Jiang, P., Chen, Y., Liu, B., He, D., Liang, C. (2019). Real-Time Detection of Apple Leaf Diseases Using Deep Learning Approach Based on Improved Convolutional Neural Networks. IEEE , 7, 59069–59080. doi: 10.1109/ACCESS.2019.2914929
Kaur, S., Pandey, S., Goel, S. (2019). Plants disease identification and classification through leaf images: A survey. Arch. Comput. Methods Eng. 26, 507–530. doi: 10.1007/s11831-018-9255-6
Krizhenvshky, A., Sutskever, I., Hinton, G. (2012).Imagenet classification with deep convolutional networks, in: Proceedings of the Conference Neural Information Processing Systems (NIPS), Lake Tahoe, NV, USA, 3–8 December. pp. 1097–1105.
Liu, W., Anguelov, D., Erhan, D., Szegedy, C., Reed, S., Fu, C., et al. (2016).SSD: Single Shot MultiBox Detector, in: Proceedings of the European Conference on Computer Vision—ECCV, Amsterdam, The Netherlands, 8–16 October. pp. 21–37.
Liu, B., Zhang, Y., He, D., Li, Y. (2018). Identification of apple leaf diseases based on deep convolutional neural networks. Symmetry 10, 11.
Mohanty, S. P., Hughes, D. P., Salathé, M. (2016). Using deep learning for image-based plant disease detection. Front. Plant Sci. 7, 1419. doi: 10.3389/fpls.2016.01419
Redmon, J., Farhadi, A. (2016). YOLO9000: Better, Faster, Stronger. IEEE Conference on Computer Vision and PatternRecognition. 2017, 6517–6525. doi: 10.1109/CVPR.2017.690
Redmon, J., Farhadi, A. (2018). Yolo V3: An incremental improvement. arXiv. arXiv:1804.02767 [cs], 1–6.
Redmon, J., Divvala, S., Girshick, R., Farhadi, A. (2016).You only look once: Unified, Real-Time Object Detection, in: Proceedings of the IEEE Conference on Computer Vision and Pattern Recognition, Las Vegas, NV, USA, 26 June–1 July. pp. 779–788.
Ren, S., He, K., Girshick, R., Sun, J. (2016). Faster R-CNN: Towards Real-Time Object Detection with Region Proposal Networks. IEEE Trans. Pattern Anal. Mach. Intell. 39, 1137–1149. doi: 10.1109/TPAMI.2016.2577031
Simonyan, K., Zisserman, A. (2014). Very deep convolutional networks for large-scale image recognition. arXiv. arXiv:1409.1556.
Sladojevic, S., Arsenovic, M., Anderla, A., Culibrk, D., Stefanovic, D. (2016). Deep neural networks based recognition of plant diseases by leaf image classification. Comput. Intell. Neurosci. 2016, 1–11. doi: 10.1155/2016/3289801
Szegedy, C., Liu, W., Jia, Y., Sermanet, P., Reed, S., Anguelov, D., et al. (2015).Going deeper with convolutions, in: Proceedings of the 2015 IEEE Conference on Computer Vision and Pattern Recognition, Boston, MA, USA, 7–12 June. pp. 1–9.
Tian, Y., Yang, G., Wang, Z., Li, E., Liang, Z. (2019). Detection of Apple Lesions in Orchards Based on Deep Learning Methods of CycleGAN and YOLOV3-Dense. J. Sens. 2019, 1–13. doi: 10.1155/2019/7630926
Wang, G., Sun, Y., Wang, J. (2017). Automatic image-based plant disease severity estimation using deep learning. Comput. Intell. Neurosci. 2017, 1–8. doi: 10.1155/2017/2917536
Xie, S., Girshick, R., Dollár, P., Tu, Z., He, K. (2017). Aggregated Residual Transformations for Deep Neural Networks. arXiv. arXiv:1611.05431.
Zheng, Y., Kong, J., Jin, X., Wang, X., Zuo, M. (2019). CropDeep: The Crop Vision Dataset for Deep-Learning-Based Classification and Detection in Precision Agriculture. Sensors 19, 1058. doi: 10.3390/s19051058
Keywords: deep learning, K-means, multiscale training, small object, object detection
Citation: Liu J and Wang X (2020) Tomato Diseases and Pests Detection Based on Improved Yolo V3 Convolutional Neural Network. Front. Plant Sci. 11:898. doi: 10.3389/fpls.2020.00898
Received: 19 December 2019; Accepted: 02 June 2020; Published: 16 June 2020.
Reviewed by:
Copyright © 2020 Liu and Wang. This is an open-access article distributed under the terms of the Creative Commons Attribution License (CC BY) . The use, distribution or reproduction in other forums is permitted, provided the original author(s) and the copyright owner(s) are credited and that the original publication in this journal is cited, in accordance with accepted academic practice. No use, distribution or reproduction is permitted which does not comply with these terms.
*Correspondence: Xuewei Wang, [email protected]
Disclaimer: All claims expressed in this article are solely those of the authors and do not necessarily represent those of their affiliated organizations, or those of the publisher, the editors and the reviewers. Any product that may be evaluated in this article or claim that may be made by its manufacturer is not guaranteed or endorsed by the publisher.

An official website of the United States government
The .gov means it’s official. Federal government websites often end in .gov or .mil. Before sharing sensitive information, make sure you’re on a federal government site.
The site is secure. The https:// ensures that you are connecting to the official website and that any information you provide is encrypted and transmitted securely.
- Publications
- Account settings
Preview improvements coming to the PMC website in October 2024. Learn More or Try it out now .
- Advanced Search
- Journal List
- Front Plant Sci
Vermicompost Improves Tomato Yield and Quality and the Biochemical Properties of Soils with Different Tomato Planting History in a Greenhouse Study
Associated data.
A greenhouse pot test was conducted to study the impacts of replacing mineral fertilizer with organic fertilizers for one full growing period on soil fertility, tomato yield and quality using soils with different tomato planting history. Four types of fertilization regimes were compared: (1) conventional fertilizer with urea, (2) chicken manure compost, (3) vermicompost, and (4) no fertilizer. The effects on plant growth, yield and fruit quality and soil properties (including microbial biomass carbon and nitrogen, NH 4 + -N, NO 3 - -N, soil water-soluble organic carbon, soil pH and electrical conductivity) were investigated in samples collected from the experimental soils at different tomato growth stages. The main results showed that: (1) vermicompost and chicken manure compost more effectively promoted plant growth, including stem diameter and plant height compared with other fertilizer treatments, in all three types of soil; (2) vermicompost improved fruit quality in each type of soil, and increased the sugar/acid ratio, and decreased nitrate concentration in fresh fruit compared with the CK treatment; (3) vermicompost led to greater improvements in fruit yield (74%), vitamin C (47%), and soluble sugar (71%) in soils with no tomato planting history compared with those in soils with long tomato planting history; and (4) vermicompost led to greater improvements in soil quality than chicken manure compost, including higher pH (averaged 7.37 vs. averaged 7.23) and lower soil electrical conductivity (averaged 204.1 vs. averaged 234.6 μS/cm) at the end of experiment in each type of soil. We conclude that vermicompost can be recommended as a fertilizer to improve tomato fruit quality and yield and soil quality, particularly for soils with no tomato planting history.
Introduction
Intensive agricultural production using inorganic fertilizers has led to increased yield, albeit at the expense of poor product quality, particularly under protected cultivation.
Tomato ( Lycopersicon esculentum ) is one of the most widely grown vegetables in Liaoning Province, where the tomato-growing area encompassed more than 85,000 hectares in 2014 (Zhao et al., 2015 ). However, determining how to improve tomato quality without reducing fruit yield remains an urgent unsolved problem. The use of organic farming with organic amendments as nutrient inputs to the soil is currently increasing, and organic farming is becoming an alternative agricultural practice to sustain economical vegetable production with minimal environmental pollution and higher fruit quality.
It is widely acknowledged that using composts and vermicomposts as amendments, rather than industrialized fertilizer and raw manure, could improve soil nutrients and promote soil health (Jack and Thies, 2006 ). Manure compost has been widely applied as it is highly accessible at low price (Hepperly et al., 2009 ; Ramirez-Guerrero and Meza-Figueroa, 2014 ), and greatly improved most of the characteristics of crop plants compared with mineral fertilizer (Da Silva et al., 2011 ). Vermicomposts are finely divided, peat-like materials produced through a non-thermophilic process involving the biodegradation and stabilization of organic materials through interactions between earthworms and microorganisms (Edwards and Burrows, 1988 ). Vermicomposts are characterized by high porosity, aeration, drainage, water-holding capacity and microbial activity. Many studies have demonstrated positive effects of vermicompost on a wide range of crops, including cereals and legumes, ornamental, and flowering plants (Chan and Griffiths, 1988 ), vegetables (Edwards and Burrows, 1988 ; Subler et al., 1998 ; Atiyeh et al., 2000 ), and field crops (Mba, 1996 ). Application of compost and vermicompost can also increase soil organic carbon, nitrates, phosphates, exchangeable calcium and some other nutrients for plants (Orozco et al., 1996 ; Garcia-Gil et al., 2000 ; Bulluck et al., 2002 ; Jindo et al., 2016 ). Most of these investigations have confirmed that manure compost and vermicompost usually has significant beneficial effects on plant growth. However, there have been very few experimental investigations exploring effects of vermicompost and manure compost applications on tomato.
Tomato-producing systems include many tomato-growing solar greenhouses with different tomato planting history. The soils with long tomato planting history cause soil degradation (such as soil acidification), soil nutrient enrichment (such as Olsen-P, total nitrogen, and available potassium; Fu et al., 2017 ), or decreased soil microbial diversity compared to the soil with short tomato planting history (Zhang et al., 2015 ). Thus, additional investigation is needed to determine whether applying vermicompost and manure compost to soils with different tomato planting history can provide the same benefits for tomato plants in terms of yield and quality.
Our main objective was to investigate the effects of vermicompost applications on tomato growth, yield and fruit quality grown in soils with different tomato planting history. The hypothesis of this study was that the effects of vermicompost on tomato yield and quality, as well as soil quality, would differ among soils with different tomato planting history due to alterations in soil traits caused. To investigate this hypothesis, a pot experiment was conducted in a greenhouse using four fertilizer treatments, including vermicompost, compost, urea, and no fertilizer, on soils with 0, 5, and 20 years of tomato planting history.
Materials and methods
Experimental site and materials.
The original soil for the experiment was collected from greenhouse tomato plants in the Guanghui township of Yuhong District, southwest Shenyang City, Liaoning Province. The soils had different years of continuous cropping: 0 (from a corn field adjacent to the tomato-planting greenhouses), 5, and 20 years.
A pot experiment was conducted from March to June of 2016 at the Greenhouse Base (123°57′ E, 41°83′ N) of Shenyang Agricultural University in Shenyang City, Liaoning Province. Polyethylene plastic pots with a diameter of 30 cm and a height of 28 cm were used. Each pot was filled with 15 kg of air-dried soil that had been passed through 1 cm sieve. The basic physical and chemical properties of the soil are shown in Table Table1. 1 . The vermicompost used in the study was obtained by adding earthworms ( Eisenia fetida ) to semi-decomposed cow manure; before use, the vermicompost was passed through a 2 mm sieve to remove the earthworms. Chicken manure compost was purchased from Ruiyuande Biotechnology Co., Ltd. (Shenyang, Liaoning Province, China). The nutrient content of the fertilizer is shown in Table Table2. 2 . The tested tomato variety, “Gold Crown No. 9,” was grown until all the fruits were harvested.
Basic characters of cultivated soil used in experiment.
Nutrient content in various manures or fertilizers.
Pot experiment design
The experiment had a randomized complete full factorial block design with two factors. Four fertilization treatments were applied to three soils with different years of continuous cropping (0, 5, and 20 years). Each treatment was repeated five times with a complete random arrangement within each block. The four fertilization treatments were CK (control: no fertilizer), urea (chemical fertilizer: 0.4 g N, 0.25 g P 2 O 5 , and 0.4 g K 2 O per kg soil that is equal to 900 kg N, 560 kg P 2 O 5 , and 900 kg K 2 O per hectare), compost (chicken manure compost), and vermicompost. A total of 8.30 g of chicken manure compost per kg soil (about 19 t chicken manure compost per hectare) and 13.10 g of vermicompost per kg soil (about 30 t vermicompost per hectare) was added to the compost and vermicompost treatments, respectively (Table (Table2). 2 ). The added amounts of compost and vermicompost were calculated according to their P concentrations due to its relatively higher content. To ensure that the concentrations of added N, P, and K were equal among the urea, compost and vermicompost treatments, additional chemical N and K were added to the latter two treatments to achieve the same N, P, and K contents as the urea treatment. Chemical N, P, and K were applied as urea, superphosphate and potassium sulfate, respectively. All the fertilizers were applied as basal fertilizer in a one-time application.
On March 12, 2016 (~35 days after seeding), when the tomato seedlings had grown to the 3- to 4-leaf stage, seedlings of a consistent size were transplanted into each pot. The plants were watered to 100% field water-holding capacity using tap water. After this watering, all the treatments received the same amount of irrigation. Soil moisture was maintained at 18–20% (w/w, i.e., 70% of field water-holding capacity) during the experiment, as determined gravimetrically, with the addition of tap water when necessary.
The pots within a block were arranged randomly in the greenhouse, with the positions re-randomized every week. The greenhouse temperature ranged from 15 to 35°C. Natural light was supplied with no supplementary light.
Soil characteristics
During the entire growth period of the tomato plants, soil samples were collected in duplicate from each pot on March 27 (seedling stage), April 26 (flowering stage), May 26 (fruiting stage), and June 25 (harvesting stage; 105 days). Part of the fresh soil was passed through a 2 mm sieve and stored at 4°C for other measurements, and the remainder of the soil was air-dried and passed through a 0.9 and a 0.15 mm sieve for the determination of physical and chemical properties.
Soil physical and chemical properties were determined as previously described (Bao, 2001 ). The soil pH (soil: water, 1:5) and electrical conductivity (EC) values (soil: water, 1:5) were measured using a Thunder Magnetic SJ-3F pH Meter (INESA, Shanghai, China) and a DDS-307 conductivity meter (INESA, Shanghai, China), respectively. The soil total carbon (TC), total nitrogen (TN) and C:N ratio were determined using an elemental analyzer (Elementar III, Germany).
The soil microbial biomass carbon (MBC) and microbial biomass nitrogen (MBN) were determined according to Brookes et al. ( 1985 ) and Vance et al. ( 1987 ) for chloroform fumigation and potassium sulfate extraction. Each fresh soil sample (equivalent to ~20 g of air-dried soil) was fumigated for 24 h at 25°C in the dark in a vacuum desiccator with ethanol-free chloroform. At the same time, each sample was fumigated without ethanol-free chloroform under the same conditions as a control. All the soil samples were extracted with 0.5 M K 2 SO 4 (1/4 w/v). The MBC and MBN contents in the extract were measured with a Multi N/C ®; 3100 analyzer (Analytik Jena, Germany) and were calculated using calibration factors of 0.45 (Vance et al., 1987 ) and 0.54 (Brookes et al., 1985 ), respectively. Water-soluble organic carbon (WSOC) was determined according to Chantigny et al. ( 2010 ). Each soil sample (~5 g of air-dried soil) was added to 45 mL of ultrapure water, shaken at 180 r/min for 1 h, and centrifuged at 4,000 r/min for 15 min. The supernatant was then passed through a 0.45 μm filter, and the filtrate was stored at 4°C until use. The WSOC contents in the filtrate were quantified using a Multi N/C® 3100 analyzer (Analytik Jena, Germany). Nitrate nitrogen ( NO 3 - -N) and ammonium nitrogen ( NH 4 + -N) were extracted from the soil using 0.01 mol/L CaCl 2 (10:1 v/w), and the NO 3 - -N and NH 4 + -N contents in the extract were measured using an AA3 Continuous-Flow Analyzer (Bran+Luebbe, Germany).
Plant parameters
Each tomato fruit was weighed, and the total yield per plant was calculated. The plant height, stem diameter and leaf chlorophyll content (SPAD) were measured at 15 days (March 27), 45 days (April 26), 75 days (May 26), and 105 days (June 25) after transplanting. The chlorophyll content of the leaves at the same part of the second branch of the plant was measured using the CCM-200 Plus Chlorophyll Analyzer (OPTI-SCIENCES, USA), and the average of three measurements was calculated. The chlorophyll content measurement was based on the difference in the light absorption rate at 653 and 931 nm.
Fruit quality was determined in tomatoes of similar color when the second ear fruit reached at 75–85% maturity (Li, 2000 ; Bao, 2001 ). The vitamin C content was determined using 2, 6-dichlorophenolindophenol and was expressed in milligrams of vitamin C per 100 g of fresh sample (mg/100 g). The total acidity was determined using the neutralization titration method and was expressed as a percentage (%). The soluble sugar contents were determined using the cyanide iodine method and expressed as a percentage (%). The nitrate contents were measured using salicylic acid colorimetry and expressed in mg/kg.
Data analysis
The data was presented as the arithmetic mean values with standard errors. One-way ANOVA was performed to compare the effects of different fertilization treatments on tomato yield and fruit quality. Two-ways ANOVA was done on stem diameter, plant height, SPAD, NH 4 + -N, NO 3 - -N, MBC, MBN, TC, TN, WSOC, EC, and pH on different soil. Differences at the 5% significance level were compared using Tukey's Honestly Significant Difference (HSD) test. ANOVA was performed on stem diameter, plant height and SPAD values, and the means were compared using Tukey's HSD test at the 5% significance level. ANOVA was used to analyze the interaction between fertilizer treatments and sampling days. A simple Pearson's correlation analysis was performed to correlate NH 4 + -N, NO 3 - N, and water-soluble carbon contents with plant growth parameters and fruit quality. The statistical analyses were performed with SPSS software, version 20.0 (IBM Corp., Armonk, NY, USA).
Plant growth and SPAD
The stem diameter, plant height and SPAD values were influenced by the fertilizer treatment in each type of soil (Table (Table3 3 and Figure Figure1; 1 ; P < 0.001). There was no interaction for these three parameters between sampling days and fertilizer treatments (Table (Table3), 3 ), indicating that fertilizer treatment had a consistent effect on these parameters. The stem diameter differed significantly among the treatments: in soil with 0 cropping years, the effect ranking was vermicompost ≥ compost ≥ urea ≥ CK; after 5 or 20 continuous cropping years, the effect was vermicompost ≥ compost > urea ≥ CK. The plant height also differed significantly: in soil with 0 cropping years, the effect ranking was vermicompost ≥ compost ≥ urea > CK; after 5 or 20 continuous cropping years, the effect was vermicompost ≥ compost > urea > CK. Moreover, SPAD values differed significantly among the treatments: in soil with 0 cropping years, the effect ranking was vermicompost ≥ compost > urea ≥ CK; after 5 or 20 continuous cropping years, the effect was vermicompost ≥ compost > urea > CK (Table (Table3 3 ).

Effects of fertilizers and sampling time (days after transplanting) on stem diameter (A) plant height (B) and SPAD (C) on soils with 0, 5, and 20 years of tomato planting history.
Results of analysis of variance ( P -values) for stem diameter, plant height, and SPAD treated with different fertilizers on soils at four sampling times after transplanting with different tomato planting history.
F-values are shown .
Yield and fruit quality
There were significant effects of fertilizers on tomato yield (Figure (Figure2A). 2A ). In soil with 0 cropping years, the treatment of vermicompost had the highest tomato yield (1,642 g/plant; followed by that of the compost treatment, 1,616 g/plant), which was significantly higher than that of the urea treatment (1,268 g/plant) and the CK treatment (956 g/plant; Figure Figure2A); 2A ); after 5 cropping years, the tomato yield of the compost treatment and the vermicompost treatment had the similar value (1,697 and 1,654 g/plant, respectively), followed by that of the urea treatment and CK treatment (1,340 and 1,169 g/plant, respectively; Figure Figure2A); 2A ); the tread of tomato yield in different fertilizer treatments kept the same after 20 cropping years (Figure (Figure2A 2A ).

Effect of fertilizers on tomato yield (A) , sugar acid ratio (B) , nitrate concentration (C) and vitamin C (D) on soils with 0, 5, and 20 continuous tomato planting years. Means in the same soil followed by different letters denote significant differences according to Tukey test ( P < 0.05).
Different fertilizers had significant effects on tomato quality, including the sugar/acid ratio, nitrate concentration and vitamin C concentration in fruit (Figures 2B–D ). Sugar/acid ratios in the treatment of vermicompost had the highest value in the each type of soil, significantly higher than that in the treatment of urea (Figure (Figure2B). 2B ). In each type of soil, the treatment of compost and vermicompost had the relatively lower the fruit nitrate concentration compared with that in the treatment of CK and urea. Particularly in the soil with 0 years tomato plant history, nitrate concentration of fruit in the vermicompost treatment was significantly lower than that in the CK treatment (67 vs. 100 mg/kg; Figure Figure2C). 2C ). Compared with the CK, all the three fertilizer application improved vitamin C content, but only the vermicompost significantly improved vitamin C content in the each type of soil (Figure (Figure2D 2D ).
Soil nitrogen
Different fertilizers, sampling days and their interaction had significant effects on soil NH 4 + -N and NO 3 - -N (Table (Table4). 4 ). An examination of the data presented in Figures Figures3A 3A , B shows that the amounts of soil NH 4 + -N, and NO 3 - -N were greater 15 days after treatment of the soil with fertilizers compared with those in the CK treatment. In most cases, the soil NH 4 + -N content followed the following order: compost ≥ vermicompost > urea > CK; the soil NO 3 - -N content followed the order: vermicompost ≥compost > urea > CK.

Effects of fertilizers and sampling time (days after transplanting) on ammonium-N (A) and nitrate-N (B) of soils on soils with 0, 5, and 20 years of tomato planting history.
Results of analysis of variance ( P values) for NH 4 + -N, NO 3 - -N, microbial biomass carbon (MBC), microbial biomass nitrogen (MBN), total nitrogen (TN), water soluble organic carbon, electric conductivity, and pH at four sampling days on soils with different tomato planting history.
Soil EC and pH
In all three types of soil, both soil EC and pH were influenced by sampling time, fertilizer treatment, and the interaction between them (Table (Table4 4 and Figure 5 ). Overall, compared with CK treatment, the other fertilizer treatments (particularly compost and urea) largely promoted soil EC at the beginning of the experiment. Over time, soil EC decreased to a much lower level than at the beginning ( Figure 5A ). At the final sampling period, after the entire growth stage, soil EC exhibited the following order: compost > vermicompost > urea > CK, regardless of years of continuous cropping.
Soil pH was highest in the CK group at almost every stage and cropping span, whereas urea treatment produced the lowest soil pH at almost every stage in soil with 0 and 20 years cropping history ( Figure 5B ). In soil with 0 cropping years, treatment with urea, compost and vermicompost produced average (from four sampling times) reductions in soil pH of −0.45, −0.27, and −0.26, respectively, compared with CK treatment; after 5 cropping years, the reduction was −0.46, −0.42, and −0.42, respectively, compared with CK treatment; and after 20 cropping years, the reduction was −0.59, −0.23, and −0.14, respectively, compared with CK. At the final growth stage, soil pH exhibited the following orders: urea ≤ compost < vermicompost < CK after 0 cropping years; compost ≤ vermicompost < urea < CK after 5 cropping years; and urea < compost < CK < vermicompost after 20 cropping years.
Water-soluble organic carbon in soil
Different fertilizer treatments, sampling days and their interaction had significant effects on the soil water-soluble organic carbon content at all four growing periods (Table (Table4). 4 ). In most of cases, the compost treatment had the highest the soil water-soluble organic carbon content (Figure (Figure4). 4 ). In the each types of soil, averaged cross sampling days, the soil water-soluble organic carbon content followed the following order: compost > vermicompost > urea > CK.

Effects of fertilizers and sampling time (days after transplanting) on water soluble organic carbon of soils on soils with 0, 5, and 20 years of tomato planting history.

Effects of fertilizers and sampling time (days after transplanting) on electrical conductivity (A) and pH (B) of soils on soils with 0, 5, and 20 years of tomato planting history.
Effects of vermicompost on fruit yield and fruit quality among soils with different tomato planting history
Table 6 shows differences in the effects of vermicompost on fruit yield and fruit quality in soils with different years of continuous cropping. The increases (%) in the yield, sugar/acid ratio, vitamin C and soluble sugar of fruit were the highest in soil with 0 cropping years. These differences were significantly higher than those after 20 cropping years while the differences after 5 cropping years were between 0 and 20 years. Furthermore, the decrease (%) in nitrate and organic acids in soil with 0 cropping years were the highest among the three soil treatments.
Correlations between soil parameters and yield and quality
A correlation matrix among the different yields, quality parameters, and soil parameters found in the present study is presented in Table Table5. 5 . The correlation matrix showed that tomato yield had a significant and positive correlation with nitrogen nutrient availability under all the fertilizer treatments ( NH 4 + -N: r = 0.459, P < 0.01; NO 3 - -N: r = 0.447, P < 0.01). Vitamin C, soluble sugar, acid content and sugar/acid ratio were significantly correlated with the water-soluble organic carbon, NH 4 + -N and NO 3 - -N in the soil.
Correlations tomato yield and quality with soil quality indicators of the different fertilizer treatments.
r-value was shown .
Effects of vermicompost on tomato growth and yield in soils with different continuous growth history
Higher plant height and thicker stem diameter were found after the treatments with vermicompost, which is consistent with previous research showing that crop plants had increased height after vermicompost was applied (Kmet'ova and Kovacik, 2013 ). This result could be due to the higher nitrogen content in soil caused by applying vermicompost in this experiment (Figure (Figure3). 3 ). Singh and Varshney ( 2013 ) found that soil NH 4 + -N and NO 3 - -N were immediately improved after applying vermicompost. Vermicompost can also enhance the growth of nitrogen-fixing microorganisms in the rhizosphere, which enhances N availability by making biologically fixed N available through the intimate mixing of ingested particles with soil (Mackay et al., 1982 ). Later, Arancon et al. ( 2003 ) indicated the improvements in crop growth and increase in fruit yields could also be due to partially to large increase in soil microbial biomass after application of vermicompost, leading to the more hormones or humate content in the vermicompost treatment. We did find increased microbial biomass carbon and nitrogen in the vermicompost (Figure S1 ). Thus, a high nutrient or hormones status of soil with vermicompost may improve the speed of tomato growth. Moreover, higher SPAD values were found in plants treated with vermicompost and compost than those in CK and urea treatment (Figure (Figure1C). 1C ). The correlation between the leaf chlorophyll content index and plant nitrogen content has been demonstrated to be useful for estimating plant nitrogen status (Li et al., 2009 ; Cabangon et al., 2011 ; Yuan et al., 2016 ). SPAD values accurately reflect the quantity of mineral N required by plants (Van Den Berg and Perkins, 2004 ). SPAD values could also demonstrate that vermicompost and compost improve the soil N status and thus are taken up by plants. Manh and Wang ( 2014 ) found that vermicompost had strongly positive effects on the seedling quality and growth of muskmelon ( Cucumis melo L.). Singh and Chauhan ( 2009 ), applied the vermicompost to the French bean ( Phaseolus vulgaris ), finding that the vermicompost promoted the germination of seeds, the height of the plant, the number of leaves of each plant, length of leaves and width of leaves of each plant and the yield. Thus, applying organic fertilizer produced an improvement in plants even at the beginning of the growth cycle. The data analysis confirmed that the final tomato yield was correlated with soil N status (Table (Table5). 5 ). Lastly, it was found that applying Ca fertilizer could improve about 10% of yield (Qin et al., 2008 ), that may be due to nutrient of Ca plays a key role in fruit growth and development (Kadir, 2005 ). In our experiment, both vermicompost and compost contains substantial Ca content (Table (Table1), 1 ), which could be a reason for relatively high yield in these treatments.
The available nutrient content in soil increased with the increasing continues tomato-planting years (Table (Table1 1 and Figure Figure3); 3 ); however, the increased available N content did not lead to an increased tomato yield among soils with different years (Figure (Figure2). 2 ). We speculated two reasons which could explain this phenomenon. Firstly, the available Ca content in soils decreased with the increasing continues tomato-planting years (Table (Table1). 1 ). Lack of available Ca may be the limiting factor for the yield in the soil with 5 or 20 years of tomato planting history (Kadir, 2005 ). Secondly, the long-term cultivation of a single crop may lead to the accumulation of harmful microbes, and the diversity and number of beneficial bacteria may decrease with the increasing continuous cropping years (Zheng et al., 2005 ). The diversity of the soil microbial community gradually decreased with the increase of the continuous monoculture tomato in a solar greenhouse (Fu et al., 2017 ). Despite that the obvious symptom was not observed in our experiment, it was still possible that the accumulative harmful microbes in the long tomato-planting history could inhibit tomato' growth and yield. The test on the microbial diversity, particularly the richness and abundance of pathogens, will be needed in the future research.
Effects of vermicompost on tomato quality in soils with different continuous growth history
The results of this study showed that tomato quality was significantly influenced by fertilizer treatments, regardless of the number of years of continuous cropping. The sugar/acid ratio and nitrate content were higher and lower, respectively, in the vermicompost-treated plants than in plants with the other fertilizer treatments, in consistent with the results of Yang et al. ( 2015 ). For cucumber, the addition vermicompost or vermicompost with organic-inorganic mixed fertilizers significantly improved the overall quality of cucumber, including the ratio of sugar to organic acid, vitamin C and soluble protein in greenhouse compared with addition of pure inorganic fertilizer and pure chick manure compost (Zhao et al., 2010 ). Vermicompost is rich in potassium (Hanc and Vasak, 2015 ; Mondal et al., 2015 ), and Çolpan et al. ( 2013 ) found that potassium improved the yield and fruit quality of tomato. Thus, the tomato quality improvement observed in the present work could be due to the increased potassium. Moreover, water-soluble organic carbon was enhanced by the addition of organic fertilizer (Figure (Figure4). 4 ). Qualities such as the vitamin C and soluble sugar contents in fruit were significantly positively correlated with water-soluble organic carbon (Table (Table5), 5 ), indicating that tomato fruit quality could be improved by adding vermicompost. Some studies have reported that tomato fruit quality can benefit from increased soil organic carbon (Jindo et al., 2016 ). In addition, Ca could also improve fruit quality (Kadir, 2005 ), and applying mixed micronutrient fertilizer (including Ca and Mg) even could reduce about 20% nitrate concentration in tomato fruit (Qin et al., 2008 ), which may explain that tomato in the treatment of vermicompost has relatively good fruit quality. Finally, different types of phytohormones have been found in vermicomposts (Zhang et al., 2014 ; Scaglia et al., 2016 ), and these phytohormones can significantly improve fruit quality. The use of organic fertilizer was shown to increase soil organic carbon and soil fertility, consequently resulting in a larger yield trend compared to a balanced chemical fertilizer (Gong et al., 2011 ). The correlations found in this study (Table (Table5) 5 ) also imply a significant positive correlation between fruit quality, water-soluble organic carbon and soil N status.
Effects of vermicompost on electrical conductivity in soils with different continuous growth history
The results of the present study show that vermicompost and compost had a significant impact on soil microbial C and N values (Figure S1 ), which are directly related to a suitable biological indicator of soil quality (Rice et al., 1996 ). Beneficial effects occurred even when the organic amendments were applied after 2 weeks (Figure (Figure1), 1 ), suggesting that applying organic fertilizer in a more sustainable production system could significantly improve soil fertility in just one tomato-growing period while improving the tomato yield to a level comparable to that of inorganically fertilized tomato. In particular, the long-term application of organic fertilizer can markedly improve soil quality (Jindo et al., 2016 ). However, due to the higher ions contained in chick manure compost (Table (Table2), 2 ), applying compost led to higher electrical conductivity compared with vermicompost, particularly during the early growing period (Figure 5) , resulting in higher electrical conductivity even at the end of the growing period. Hashemimajd et al. ( 2004 ) compared vermicompost and some types of composts finding that vermicompost had the lowest electrical conductivity. Lazcano et al. ( 2009 ) even found that excessively applying composts could lead to tomato plants death due to the high concentrations of certain ions in composts and claimed that the dosage of application compost needs to be well controlled. Thus, even chick manure compost improved tomato yield and quality in this study (Figure (Figure2), 2 ), but did harm soil more than vermicompost in the way of enhancing soil electrical conductivity.
In conclusion, our results suggest that vermicompost can improve the biochemical properties of soil under different years of continuous growth, thereby increasing tomato growth, yield, and fruit quality compared with urea. Moreover, considering the higher electrical conductivity and lower soil pH achieved by applying compost, vermicompost could be a better recommendation for soils. Especially for the new soil without tomato planting history, vermicompost can produce better improvements in fruit yield and quality compared with old tomato-planting soils (Table (Table6). 6 ). However, field studies are still needed to confirm our greenhouse results. These studies should be designed to elucidate the impacts of organic fertilizers on soil microbial processes and nutrient cycling on different soil types, to increase tomato yields under sustainable production systems. The final goal is to optimize fertilizer management to maximize yields and quality while reducing the use of inorganic fertilizer and maintaining good-quality soil.
Increased or decreased percentage in fruit yield or fruit quality in vermicompost treatment compared with CK on each soil.
“(Vermicompost – CK)/CK * 100” was used here. Means (±SE) followed by different letters denote significant differences according to Tukey test (P < 0.05) among three soil treatments under each column .
Author contributions
X-XW analyzed the data, wrote the first manuscript and modified it. FZ performed the experiment, collected the samples and collected the data. GZ and YZ assisted to manage the experiment and collect the data. LY designed the experiments, revised the manuscript and applied funding to support the study. All the authors discussed the results and commented on the manuscript.
Conflict of interest statement
The authors declare that the research was conducted in the absence of any commercial or financial relationships that could be construed as a potential conflict of interest.
Acknowledgments
We are grateful to the research grants from the National Natural Science Foundation of China (31372132), the National Key Research and Development Program of China (2016YFD0201004) and the Specialized Research Fund for the Doctoral Program of Chinese Higher Education (20132103110008).
Supplementary material
The Supplementary Material for this article can be found online at: https://www.frontiersin.org/articles/10.3389/fpls.2017.01978/full#supplementary-material
- Arancon N. Q., Edwards C. A., Bierman P., Metzger J. D., Lee S., Welch C. (2003). Effects of vermicomposts on growth and marketable fruits of field-grown tomatoes, peppers and strawberries: the 7th international symposium on earthworm ecology · Cardiff · Wales · 2002 . Pedobiologia 47 , 731–735. 10.1078/0031-4056-00251 [ CrossRef ] [ Google Scholar ]
- Atiyeh R., Subler S., Edwards C., Bachman G., Metzger J., Shuster W. (2000). Effects of vermicomposts and composts on plant growth in horticultural container media and soil . Pedobiologia 44 , 579–590. 10.1078/S0031-4056(04)70073-6 [ CrossRef ] [ Google Scholar ]
- Bao S. (2001). Analysis of Soil Agro-Chemistry . Beijing: Agricultural Press Chinese. [ Google Scholar ]
- Brookes P., Landman A., Pruden G., Jenkinson D. (1985). Chloroform fumigation and the release of soil nitrogen: a rapid direct extraction method to measure microbial biomass nitrogen in soil . Soil Biol. Biochem. 17 , 837–842. 10.1016/0038-0717(85)90144-0 [ CrossRef ] [ Google Scholar ]
- Bulluck L., Brosius M., Evanylo G., Ristaino J. (2002). Organic and synthetic fertility amendments influence soil microbial, physical and chemical properties on organic and conventional farms . Appl. Soil Ecol. 19 , 147–160. 10.1016/S0929-1393(01)00187-1 [ CrossRef ] [ Google Scholar ]
- Cabangon R. J., Castillo E. G., Tuong T. P. (2011). Chlorophyll meter-based nitrogen management of rice grown under alternate wetting and drying irrigation . Field Crops Res. 121 , 136–146. 10.1016/j.fcr.2010.12.002 [ CrossRef ] [ Google Scholar ]
- Chan P. L., Griffiths D. (1988). The vermicomposting of pre-treated pig manure . Biol. Wastes 24 , 57–69. 10.1016/0269-7483(88)90027-4 [ CrossRef ] [ Google Scholar ]
- Chantigny M. H., Curtin D., Beare M. H., Greenfield L. G. (2010). Influence of temperature on water-extractable organic matter and ammonium production in mineral soils . Soil Sci. Soc. Am. J. 74 , 517–524. 10.2136/sssaj2008.0347 [ CrossRef ] [ Google Scholar ]
- Da Silva T. R., Menezes J. F. S., Simon G. A., De Assis R. L., Santos C. J. D., Gomes G. V. (2011). Corn cultivation and availability of phosphorus under fertilization with chicken manure . Rev. Bras. Eng. Agric. E Ambiental 15 , 903–910. 10.1590/S1415-43662011000900005 [ CrossRef ] [ Google Scholar ]
- Edwards C., Burrows I. (1988). The potential of earthworm composts as plant growth media , in Earthworms in Waste and Environmental Management , eds Edward C. A., Neuhauser E. F. (Hague: SPB Academic Press; ), 2132. [ Google Scholar ]
- Çolpan E., Zengin M., Zbahçe A. (2013). The effects of potassium on the yield and fruit quality components of stick tomato . Hortic. Environ. Biotechnol. 54 , 20–28. 10.1007/s13580-013-0080-4 [ CrossRef ] [ Google Scholar ]
- Fu H., Zhang G., Zhang F., Sun Z., Geng G., Li T. (2017). Effects of continuous tomato monoculture on soil microbial properties and enzyme activities in a solar greenhouse . Sustainability 9 :317 10.3390/su9020317 [ CrossRef ] [ Google Scholar ]
- Garcia-Gil J., Plaza C., Soler-Rovira P., Polo A. (2000). Long-term effects of municipal solid waste compost application on soil enzyme activities and microbial biomass . Soil Biol. Biochem. 32 , 1907–1913. 10.1016/S0038-0717(00)00165-6 [ CrossRef ] [ Google Scholar ]
- Gong W., Yan X. Y., Wang J. Y., Hu T. X., Gong Y. B. (2011). Long-term applications of chemical and organic fertilizers on plant-available nitrogen pools and nitrogen management index . Biol. Fertil. Soils 47 , 767–775. 10.1007/s00374-011-0585-x [ CrossRef ] [ Google Scholar ]
- Hanc A., Vasak F. (2015). Processing separated digestate by vermicomposting technology using earthworms of the genus Eisenia . Int. J. Environ. Sci. Technol. 12 , 1183–1190. 10.1007/s13762-014-0500-8 [ CrossRef ] [ Google Scholar ]
- Hashemimajd K., Kalbasi M., Golchin A., Shariatmadari H. (2004). Comparison of vermicompost and composts as potting media for growth of tomatoes . J. Plant Nutr. 27 , 1107–1123. 10.1081/PLN-120037538 [ CrossRef ] [ Google Scholar ]
- Hepperly P., Lotter D., Ulsh C. Z., Seidel R., Reider C. (2009). Compost, manure and synthetic fertilizer influences crop yields, soil properties, nitrate leaching and crop nutrient content . Compost Sci. Util. 17 , 117–126. 10.1080/1065657X.2009.10702410 [ CrossRef ] [ Google Scholar ]
- Jack A. L., Thies J. E. (2006). Compost and vermicompost as amendments promoting soil health , in Biological Approaches to Sustainable Soil Systems , ed Uphoff N. (New York, NY: CRC Press; ), 453–466. [ Google Scholar ]
- Jindo K., Chocano C., De Aguilar J. M., Gonzalez D., Hernandez T., Garcia C. (2016). Impact of compost application during 5 years on crop production, soil microbial activity, carbon fraction, and humification process . Commun. Soil Sci. Plant Anal. 47 , 1907–1919. 10.1080/00103624.2016.1206922 [ CrossRef ] [ Google Scholar ]
- Kadir S. A. (2005). Fruit quality at harvest of “Jonathan” apple treated with foliarly-applied calcium chloride . J. Plant Nutr. 27 , 1991–2006. 10.1081/PLN-200030102 [ CrossRef ] [ Google Scholar ]
- Kmet'ova M., Kovacik P. (2013). The impact of vermicompost application on yield parameters of maize . Mendelnet 2013 , 75–81. [ Google Scholar ]
- Lazcano C., Arnold J., Tato A., Zaller J., Domínguez J. (2009). Compost and vermicompost as nursery pot components: effects on tomato plant growth and morphology . Spanish J. Agric. Res. 7 , 944–951. 10.5424/sjar/2009074-1107 [ CrossRef ] [ Google Scholar ]
- Li H. (2000). Plant Physiology and Biochemistry Principle and Techniques . Beijing: Higher Education Press. [ Google Scholar ]
- Li J. W., Yang J. P., Fei P. P., Song J. L., Li D. S., Ge C. S., et al. (2009). Responses of rice leaf thickness, SPAD readings and chlorophyll a/b ratios to different nitrogen supply rates in paddy field . Field Crops Res. 114 , 426–432. 10.1016/j.fcr.2009.09.009 [ CrossRef ] [ Google Scholar ]
- Mackay A., Syers J., Springett J., Gregg P. (1982). Plant availability of phosphorus in superphosphate and a phosphate rock as influenced by earthworms . Soil Biol. Biochem. 14 , 281–287. 10.1016/0038-0717(82)90038-4 [ CrossRef ] [ Google Scholar ]
- Manh V. H., Wang C. H. (2014). Vermicompost as an important component in substrate: effects on seedling quality and growth of muskmelon ( Cucumis melo L.) . APCBEE Proc. 8 , 32–40. 10.1016/j.apcbee.2014.01.076 [ CrossRef ] [ Google Scholar ]
- Mba C. C. (1996). Treated-cassava peel vermicomposts enhanced earthworm activities and cowpea growth in field plots . Resour. Conserv. Recyc. 17 , 219–226. 10.1016/0921-3449(96)01102-0 [ CrossRef ] [ Google Scholar ]
- Mondal T., Datta J. K., Mondal N. K. (2015). Influence of indigenous inputs on the properties of old alluvial soil in a mustard cropping system . Arch. Agron. Soil Sci. 61 , 1319–1332. 10.1080/03650340.2014.1000877 [ CrossRef ] [ Google Scholar ]
- Orozco F., Cegarra J., Trujillo L., Roig A. (1996). Vermicomposting of coffee pulp using the earthworm Eisenia fetida : effects on C and N contents and the availability of nutrients . Biol. Fertil. Soils 22 , 162–166. 10.1007/BF00384449 [ CrossRef ] [ Google Scholar ]
- Qin Y.-S., Tu S.-H., Feng W.-Q., Sun X.-F. (2008). Effects of calcium, magnesium, zinc and boron fertilizers on yield and quality of field tomatoes on Chengdu Plain (in Chinese with English abstract) . Soil Fertil. Sci. China 3 , 57–59. 10.3969/j.issn.1673-6257.2008.03.015 [ CrossRef ] [ Google Scholar ]
- Ramirez-Guerrero H., Meza-Figueroa C. (2014). Strengthening potato production and ecological transition using organic fertilization . Rev. Fac. Agron. Univers. Zulia 31 , 1–11. [ Google Scholar ]
- Rice C. W., Moorman T. B., Beare M. (1996). Role of microbial biomass carbon and nitrogen in soil quality . Methods Assess. Soil Qual. 203–215. [ Google Scholar ]
- Scaglia B., Nunes R. R., Rezende M. O. O., Tambone F., Adani F. (2016). Investigating organic molecules responsible of auxin-like activity of humic acid fraction extracted from vermicompost . Sci. Total Environ. 562 , 289–295. 10.1016/j.scitotenv.2016.03.212 [ PubMed ] [ CrossRef ] [ Google Scholar ]
- Singh N. I., Chauhan J. (2009). Response of French bean ( Phaseolus vulgaris L.) to organic manures and inorganic fertilizer on growth and yield parameters under irrigated condition . Nat. Sci. 7 , 52–54. [ Google Scholar ]
- Singh R. P., Varshney G. (2013). Effects of carbofuran on availability of macronutrients and growth of tomato plants in natural soils and soils amended with inorganic fertilizers and vermicompost . Commun. Soil Sci. Plant Anal. 44 , 2571–2586. 10.1080/00103624.2013.803568 [ CrossRef ] [ Google Scholar ]
- Subler S., Edwards C., Metzger J. (1998). Comparing vermicomposts and composts . Biocycle 39 , 63–66. [ Google Scholar ]
- Vance E., Brookes P., Jenkinson D. (1987). An extraction method for measuring soil microbial biomass C . Soil Biol. Biochem. 19 , 703–707. 10.1016/0038-0717(87)90052-6 [ CrossRef ] [ Google Scholar ]
- Van Den Berg A., Perkins T. (2004). Evaluation of a portable chlorophyll meter to estimate chlorophyll and nitrogen contents in sugar maple ( Acer saccharum Marsh.) leaves . For. Ecol. Manage. 200 , 113–117. 10.1016/j.foreco.2004.06.005 [ CrossRef ] [ Google Scholar ]
- Yang L. J., Zhao F. Y., Chang Q., Li T. L., Li F. S. (2015). Effects of vermicomposts on tomato yield and quality and soil fertility in greenhouse under different soil water regimes . Agric. Water Manage. 160 , 98–105. 10.1016/j.agwat.2015.07.002 [ CrossRef ] [ Google Scholar ]
- Yuan Z. F., Cao Q., Zhang K., Ata-Ul-Karim S. T., Tian Y. C., Zhu Y., et al.. (2016). Optimal leaf positions for SPAD meter measurement in rice . Front. Plant Sci. 7 :719. 10.3389/fpls.2016.00719 [ PMC free article ] [ PubMed ] [ CrossRef ] [ Google Scholar ]
- Zhang H., Tan S. N., Wong W. S., Ng C. Y. L., Teo C. H., Ge L., et al. (2014). Mass spectrometric evidence for the occurrence of plant growth promoting cytokinins in vermicompost tea . Biol. Fertil. Soils 50 , 401–403. 10.1007/s00374-013-0846-y [ CrossRef ] [ Google Scholar ]
- Zhang X., Tian L., Wu P., Gao Y., Li J. (2015). Changes of soil nutrients and microbial community diversity in responses to different growth environments and cultivation practices in 30 years . J. Plant Nutr. Fertil. 21 , 1581–1589. 10.11674/zwyf.2015.0625 [ CrossRef ] [ Google Scholar ]
- Zhao H., Luo J., Dan Y., Wang A., Liu P., Feng K. (2010). Effects of vermicompost organic-inorganic mixed fertilizer on yield and quality components of cucumber cultivated in greenhouse (in Chinese with English abstract) . Plant Nutr. Fertil. Sci. 16 , 1288–1293. 10.11674/zwyf.2010.0533 [ CrossRef ] [ Google Scholar ]
- Zhao Y. P., Jia Q., Qi X. H. (2015). Present situation and development countermeasure of tomato production in Liaoning Province (in Chinese) . China Vegetables 10 , 6–9. 10.3969/j.issn.1000-6346.2015.10.002 [ CrossRef ] [ Google Scholar ]
- Zheng L., Hu J., Lin C., Tang Q., Guo Q. (2005). The production of succession cropping obstacles and its prevention and cure steps (in Chinese with English abstract) . Chin. J. Trop. Agric. 2 , 58–62. 10.3969/j.issn.1009-2196.2005.02.014 [ CrossRef ] [ Google Scholar ]
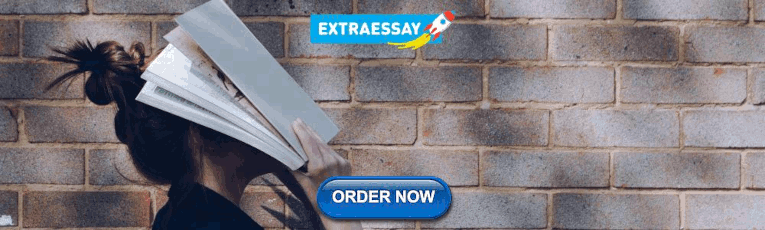
IMAGES
VIDEO
COMMENTS
Since 2000, over a thousand scientific papers per year relating to tomato research have been published, not including those in the less widely read literature, conference and workshop proceedings.
In a more recent study based on previously published original research articles, an average tomato consists of ash 8.75%, water 94.17 (g/100 g), moisture 91.18 (g/100 g), total protein 17.71 (g/100 g ... Sterols are mainly found in plants, animals, and microorganisms. Plant sterols, also known as phytosterols, commonly occur as a mixture of β ...
The research outlined in this review paper discusses potential health benefits associated with a diet enriched with tomatoes and tomato products. This includes details of previous studies investigating the anticancer properties of tomatoes, protection against cardiovascular and neurodegenerative diseases and diabetes, maintenance of a healthy ...
Tomato has been widely used not only as food, but also as research material. The tomato plant has many interesting features such as fleshy fruit, a sympodial shoot, and compound leaves, which other model plants (e.g., rice and Arabidopsis) do not have. Most of these traits are agronomically important and cannot be studied using other model ...
Tomato is the most important horticultural crop in the world. The yields for this crop are highest in Southeastern Spain. In this work we studied a commercial variety of tomato, with different ...
The tomato leaf dataset was selected and used to performe deep learning; the author used the disease classes and achieved a better accuracy rate. A simple CNN model with eight hidden layers has been used to identify the conditions of a tomato plant. The proposed techniques show optimal results compared to other classical models [32,33,34,35].
Introduction. Tomato (Solanum lycopersicum L.) is the second most important fruit or vegetable crop next to potato (Solanum tuberosum L.), with approximately 182.3 million tons of tomato fruits produced on 4.85 million ha each year (FAOSTAT, 2019).Asia accounts for 61.1% of global tomato production, while Europe, America, and Africa produced 13.5%, 13.4%, and 11.8% of the total tomato yield ...
Tomato, Solanum lycopersicum var. lycopersicum L. (SLL), is one of the most consumed vegetables all over the world with a production that exceeds 180 million tonnes (FAO, 2017). Its cultivation ...
Feature papers represent the most advanced research with significant potential for high impact in the field. A Feature Paper should be a substantial original Article that involves several techniques or approaches, provides an outlook for future research directions and describes possible research applications. ... Tomato plants responded to CO 2 ...
This Research Topic features 12 papers covering relevant subjects, including the production and processing of tomatoes and tomato-based foods and ingredients, as well as the bioaccessibility and health-promoting effects of tomato bioactive compounds. Traditional varieties represent an important component of agricultural biodiversity and play a ...
The diploid nature of tomato, genomic resources and a wide range of single gene mutants make it an excellent model plant to study dicot crop species and especially those with fleshy fruits. In this special collection, we bring together a range of papers to explore the latest developments and scientific insights in tomato molecular biology.
Tomato plants' disease detection and classification at the earliest stage can save the farmers from expensive crop sprays and can assist in increasing the food quantity. Although, extensive work ...
Tomato is the most widespread vegetable crop in the world. In Italy, tomatoes are mainly cultivated in the South and in the Campania region, precisely in the area called Agro Nocerino-Sarnese. This flatland is affected by an extreme level of environmental degradation, especially related to the Sarno River, where concentrations of Potential Toxic Elements (PTEs) have been found to be higher ...
List of tables 5 1 General perspective of tomato crop and its societal importance 7 1.1 Origin and distribution of tomato 7 1.2 Nutritional importance of the crop 8 1.3 Economic and social importance of the crop 8 1.4 Losses from the field to consumers 9 1.5 Globalization and its impact on horticultural farmers. A social point of view 10 2 Factors determining the quality of tomato fruits 12
The wild tomato plant originated from the warmer areas of South America, including Ecuador, Chile, and the Galapagos Islands (Peralta & Spooner, 2007; Robertson & Labate, 2007; Rothan, Diouf, & Causse, 2018), with Peru as its center of origin.Based on its physical characteristics, the tomato was placed alongside the potato in the genus Solanum (Linnaeus, in 1753); however, Philip Miller placed ...
Abstract. Tomato is one of the most consumed and widely grown vegetable crops in the world. It is a popular vegetable/fruit and an important source of vitamins and minerals in human diet. One ...
2.1. Plant and growth conditions. Seeds of tomato (Solanum lycopersicum Mill.) were surface sterilized in 7% sodium hypochlorite for 10 min, subsequently washed thoroughly with distilled water.The sterilized seeds were divided into four sets. Seeds of the 1st set were soaked in distilled water to serve as control, the other three sets (2nd, 3rd and 4th) were soaked in shikimic acid at 30, 60 ...
This review paper is expected to provide the requisite information in tomato value chain to ensure sustainable production of tomato globally. Determinate Tomato (A) and Indeterminate Tomato plants ...
This study addresses the challenge of timely and accurate detection of diseases in tomato plants, crucial for effective plant protection management. Conventional manual inspection methods are time-consuming and prone to errors, resulting in delays in implementing necessary protection measures.
Tomato cropping systems range from hydroponic greenhouse systems with complete ambient control to organic outdoor production. Greenhouse gas emissions (in g CO 2 equivalent per kg tomatoes) in the research area vary largely from 34 in organic outdoor production, to 63 in organic polytunnels, to 99 in conventional polytunnels, and up to 1570 in heated greenhouses [].
At site A for tomato, 55 mL organic fertilizer increased plant height, number of leaves, leaf area and stem girth relative to the control by 356.0, 97.0, 91.4, and 70.3% respectively, while for ...
The use of YoloV8 via RoboFlow is presented in this paper to detect nine (9) common tomato leaf diseases. It generated 99% average precision from both the validation and test sets.
Compared with the whole tomato plant image, the disease spots and pests are small objects. Therefore, Yolo V3 model is a little inadequate in the scale when recognizing tomato disease spots and pests. In view of this situation, Yolo V3 model is improved to adapt to specific tomato diseases and pests detection tasks.
During the entire growth period of the tomato plants, soil samples were collected in duplicate from each pot on March 27 (seedling stage), April 26 (flowering stage), May 26 (fruiting stage), and June 25 (harvesting stage; 105 days). ... We are grateful to the research grants from the National Natural Science Foundation of China (31372132), the ...