Research Report Templates
Uncover the potential of your research with our report templates. They're the missing piece in your puzzle of data!
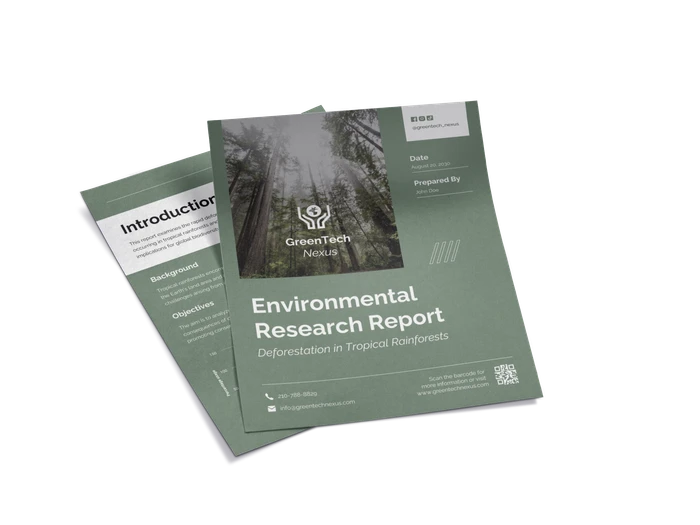
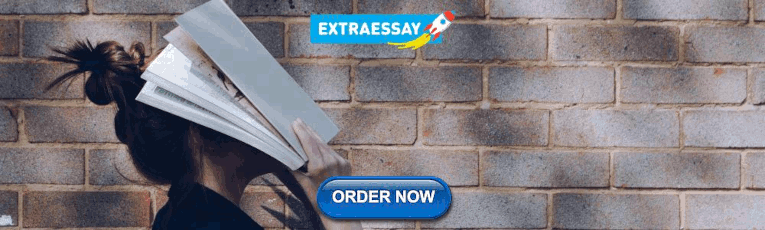
Other report templates
- Human resources
- Executive summary
- Survey results
- Project status
- Construction
Popular template categories
- Infographics
- Presentations
- White papers
- Letterheads
- Newsletters
- Business cards
- Certificates
- Invitations
- Social media
- Table of contents
- Magazine covers
- Price lists
- Album covers
- Book covers
- See All Templates
Got any suggestions?
We want to hear from you! Send us a message and help improve Slidesgo
Top searches
Trending searches
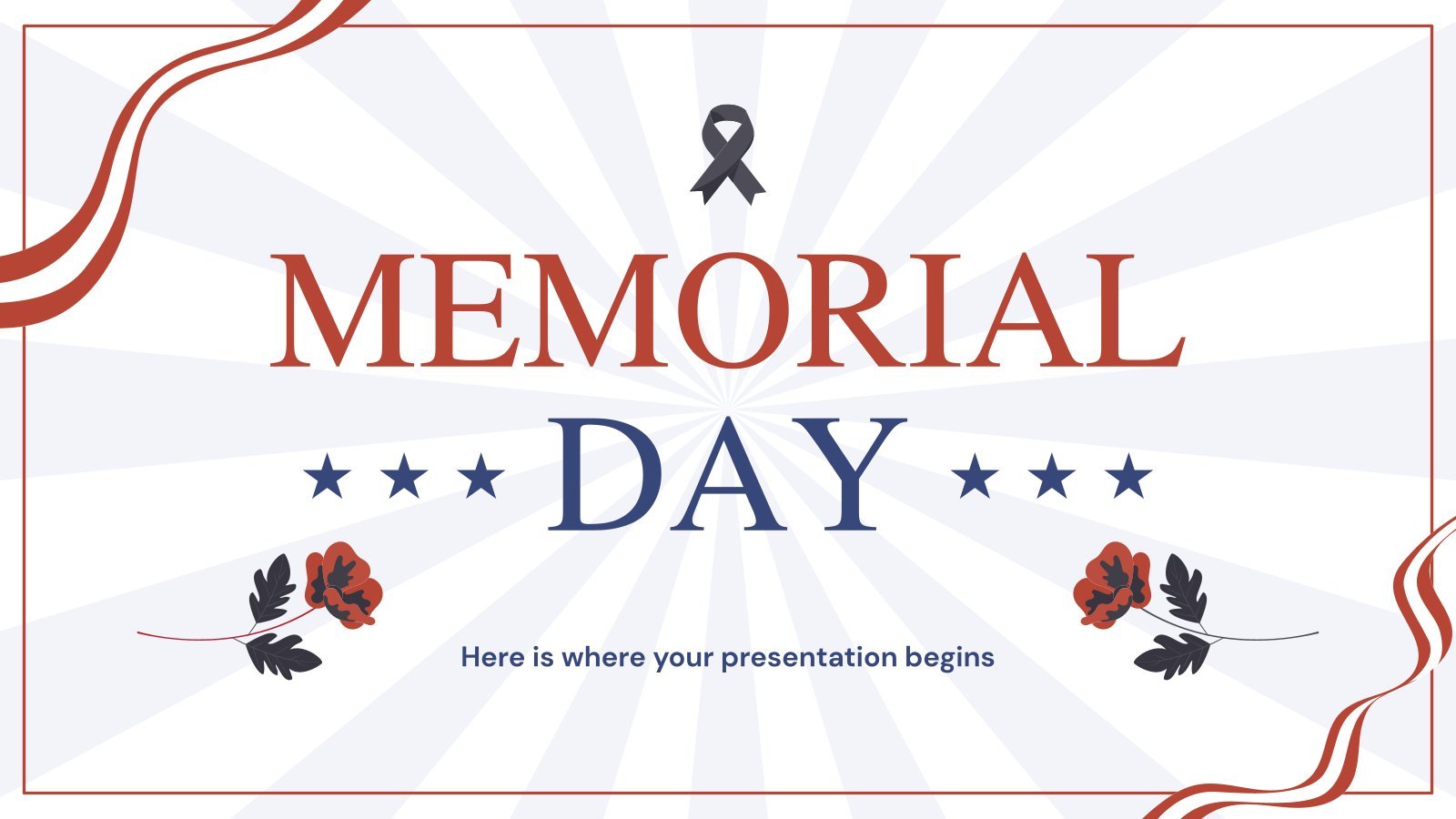
memorial day
12 templates
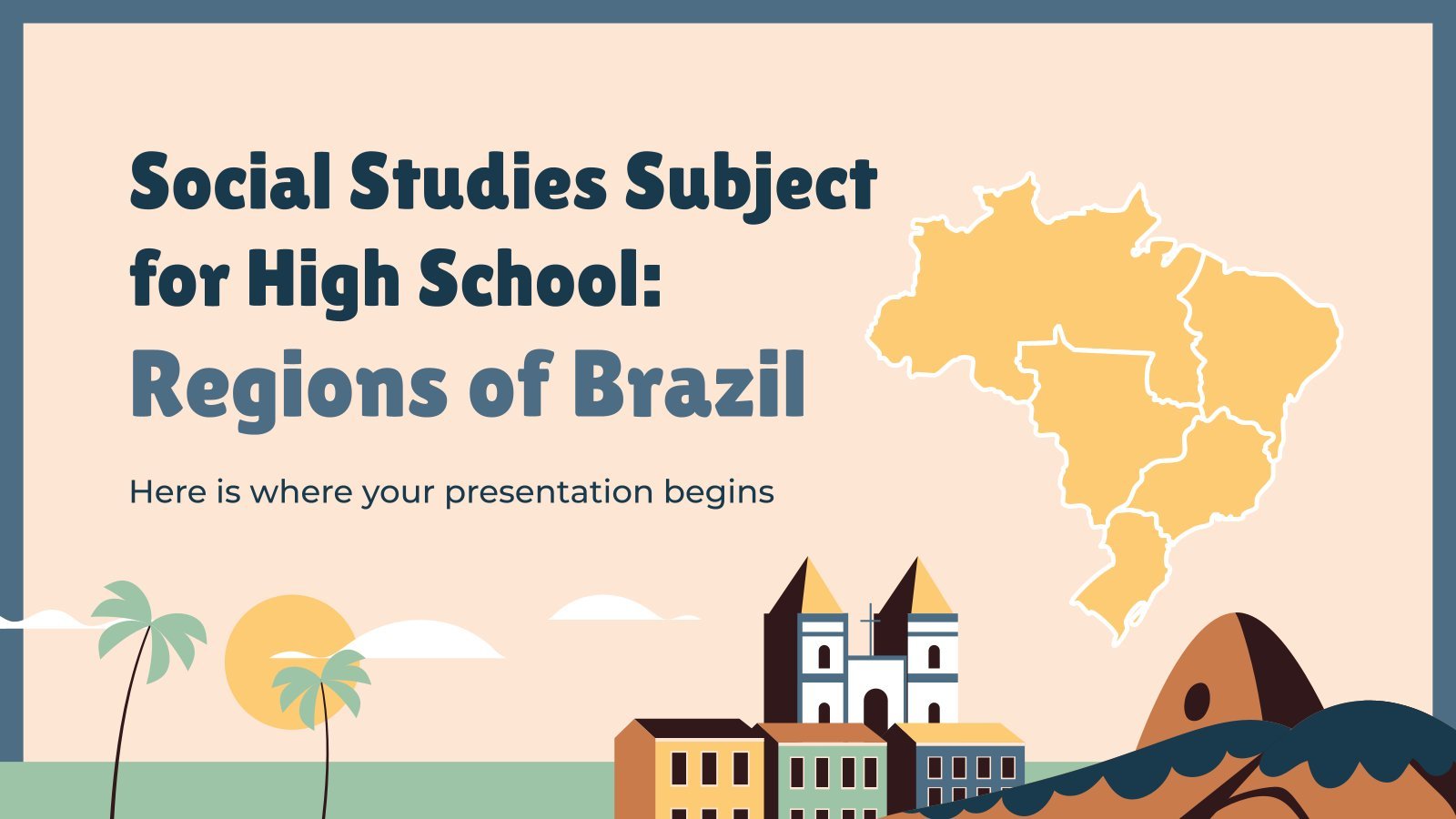
66 templates
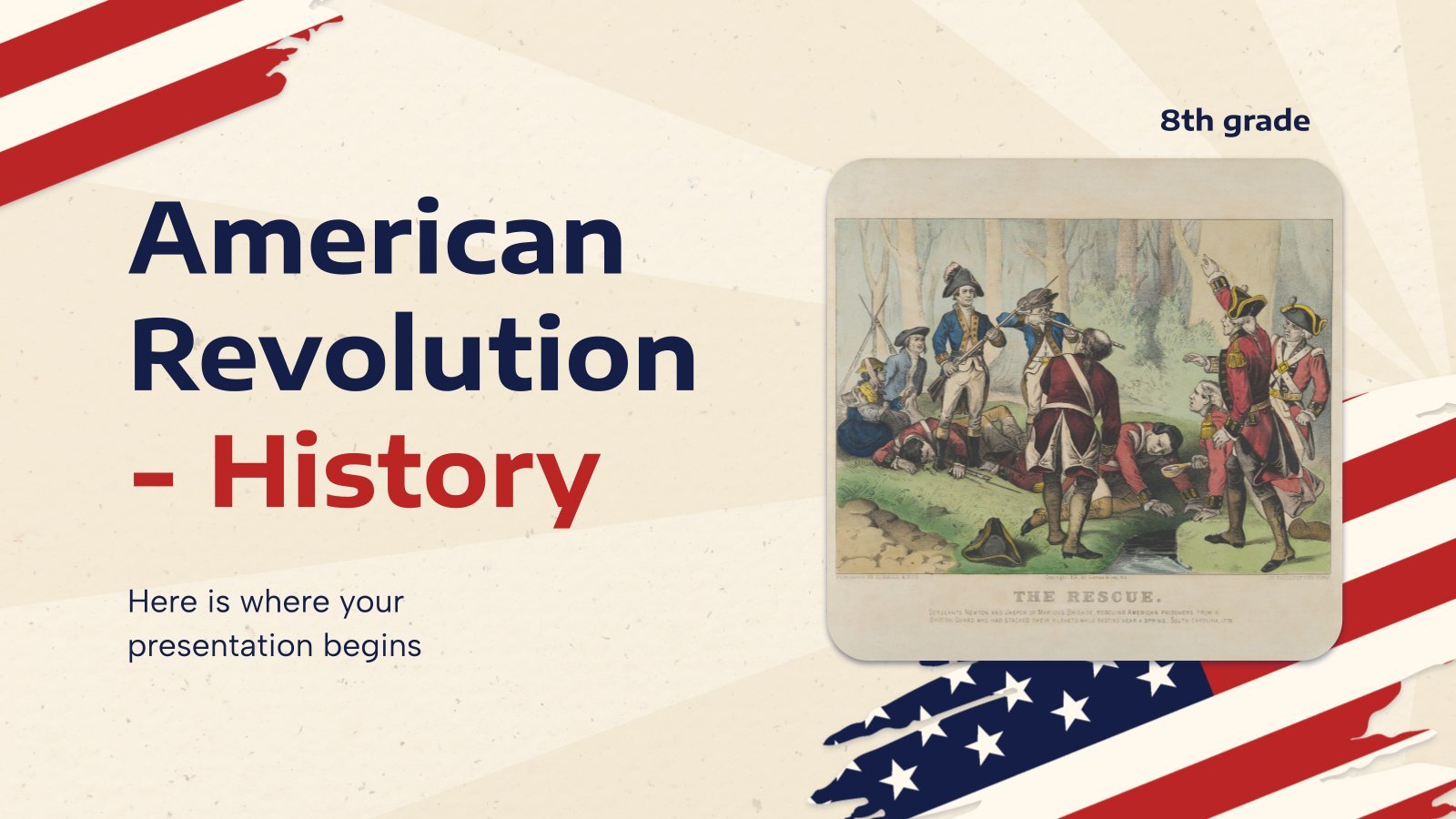
american history
75 templates
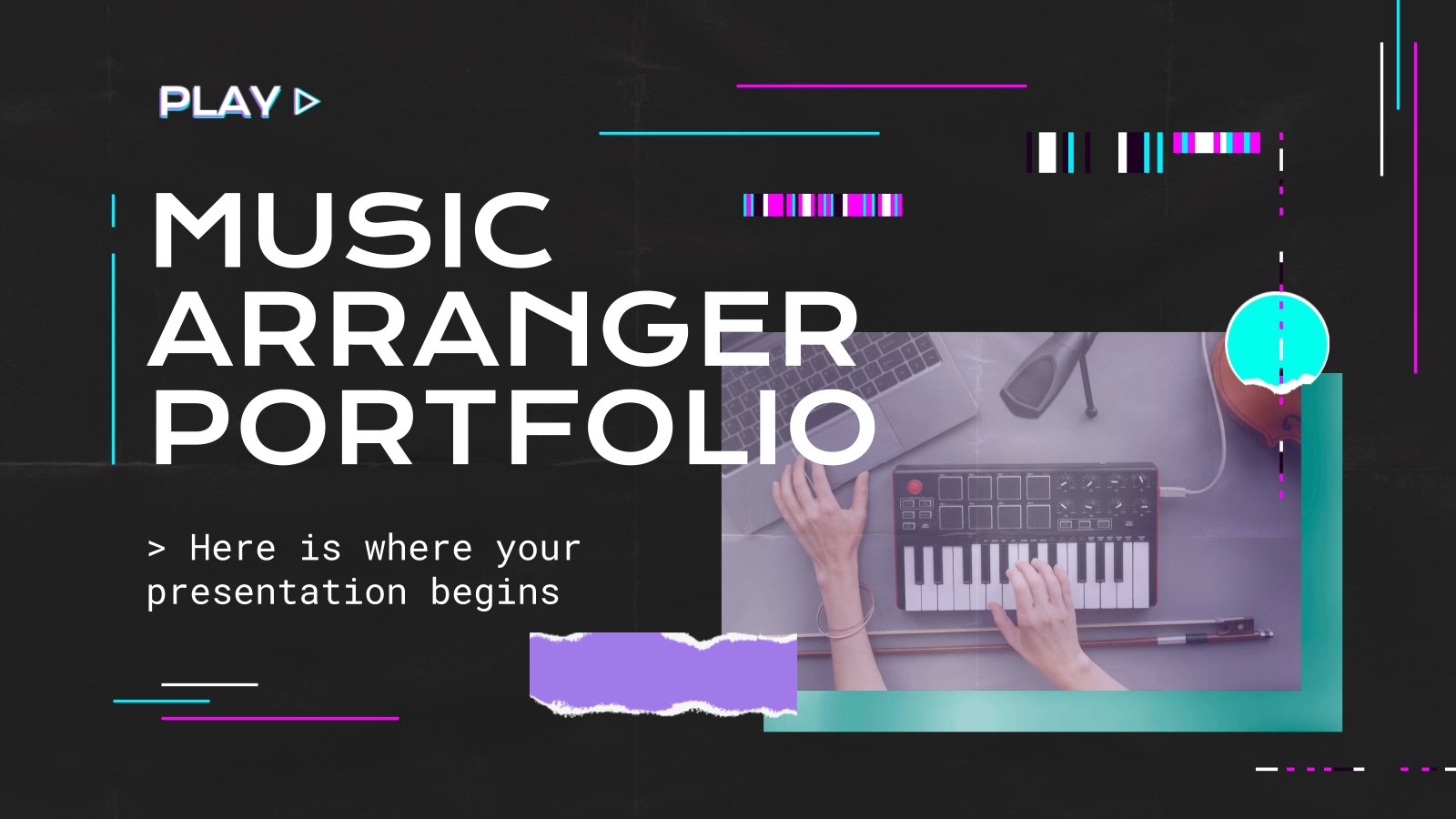
music video
21 templates
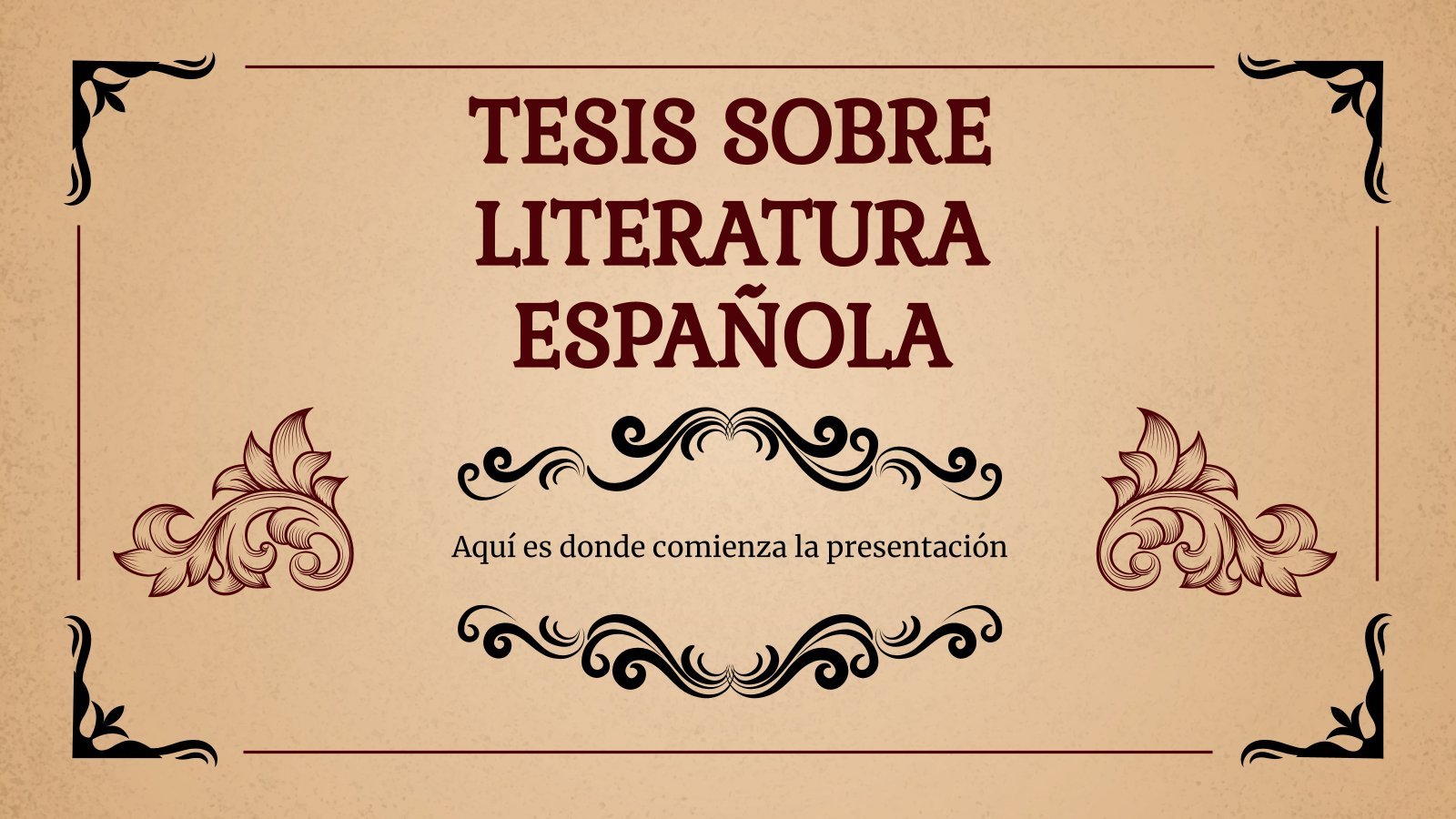
150 templates
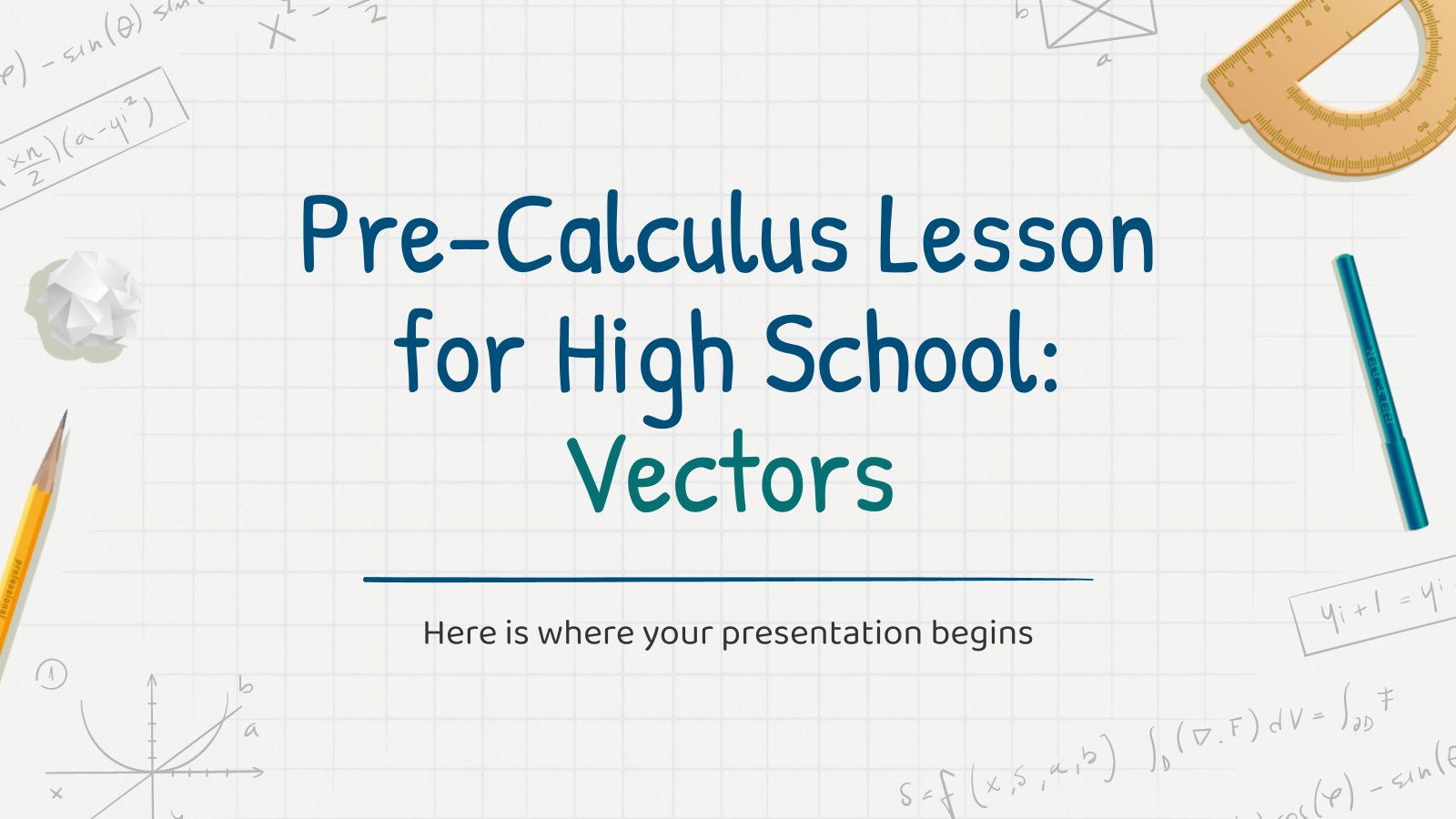
Research Presentation templates
Customize our free themes and templates for google slides or powerpoint and explain what your research is about. these designs are easy to edit, so that will speed things up.
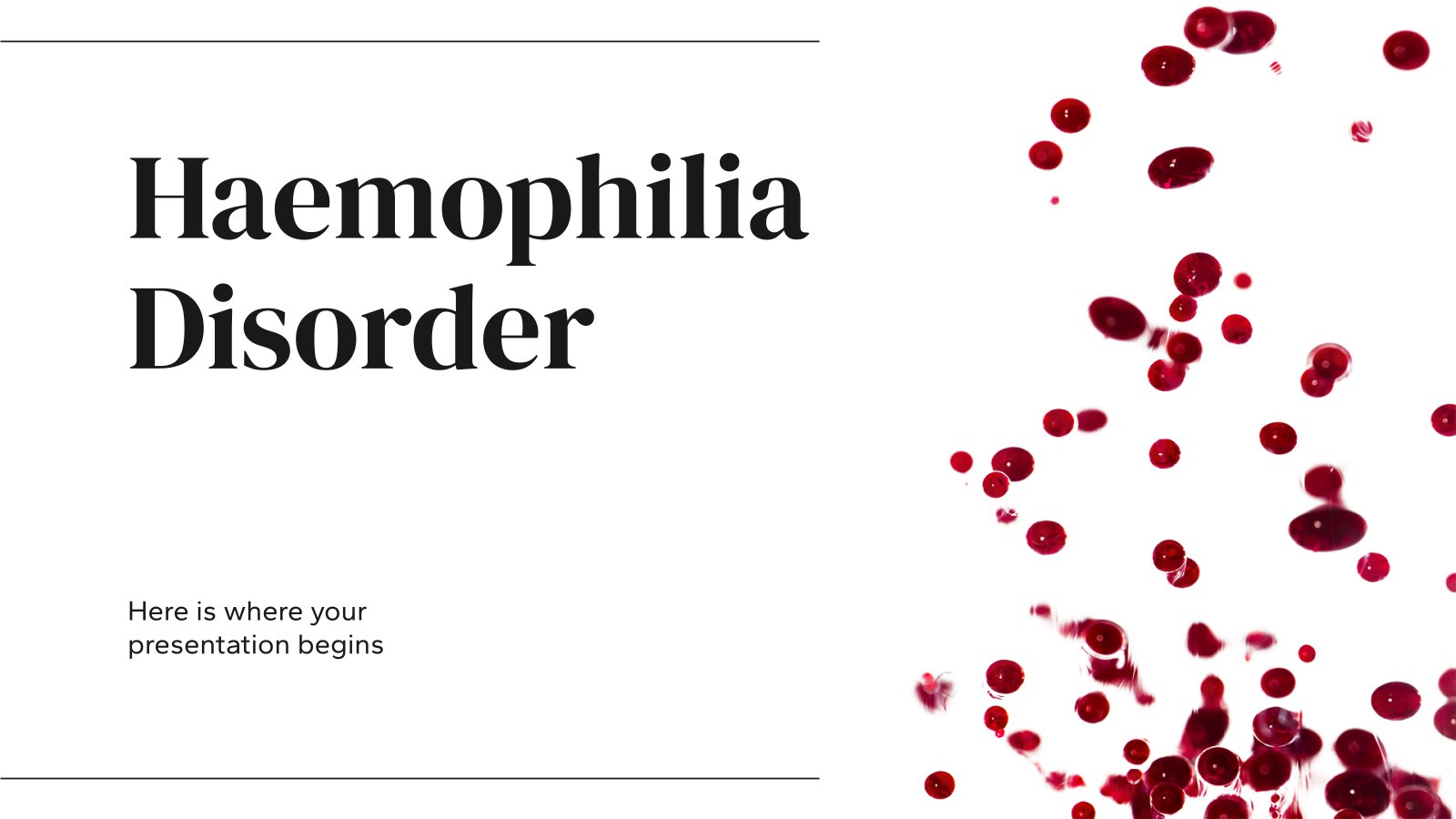
It seems that you like this template!
Haemophilia disorder.
Download the Haemophilia Disorder presentation for PowerPoint or Google Slides. Taking care of yourself and of those around you is key! By learning about various illnesses and how they are spread, people can get a better understanding of them and make informed decisions about eating, exercise, and seeking medical attention....
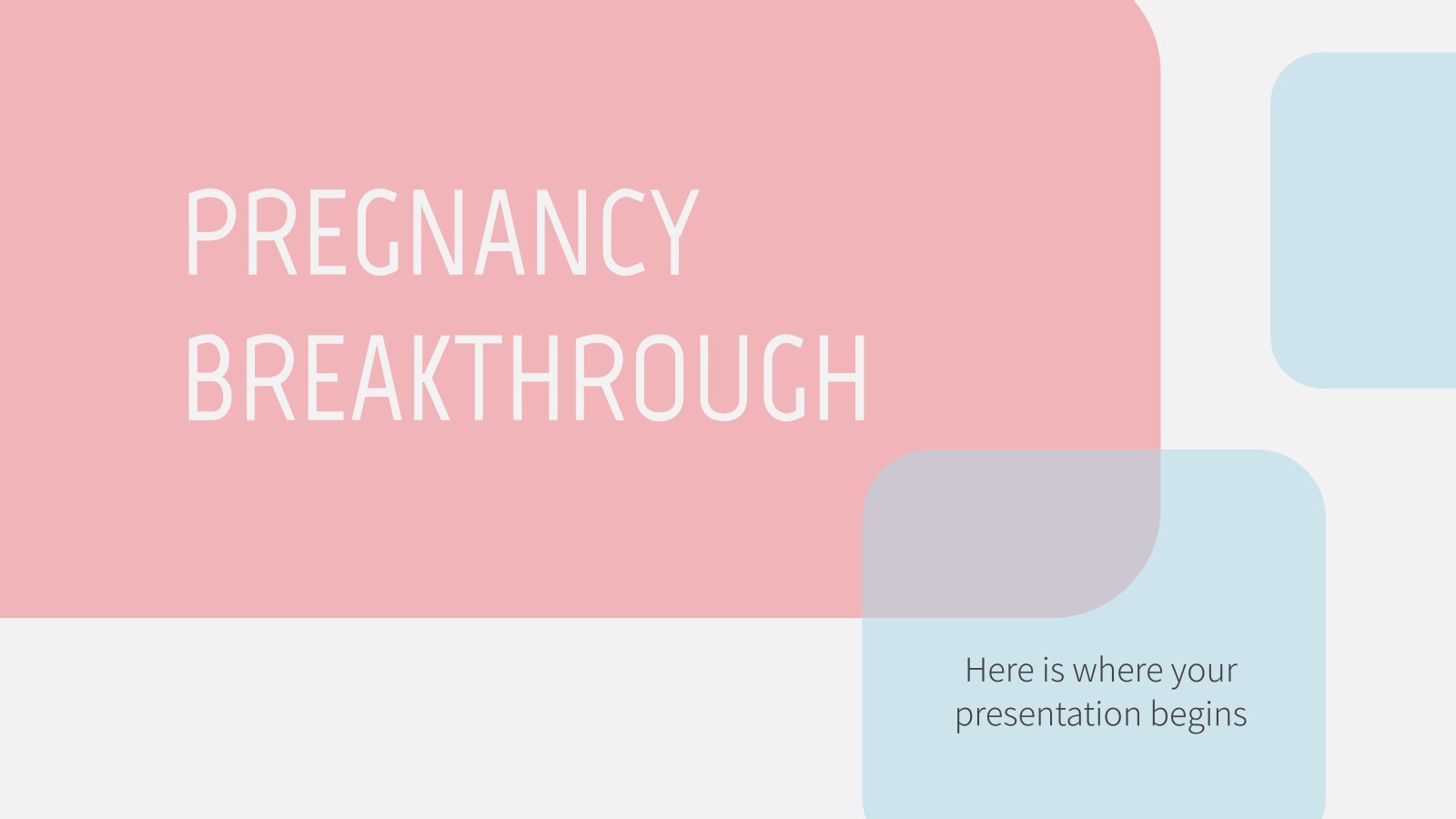
Premium template
Unlock this template and gain unlimited access
Pregnancy Breakthrough
Giving birth to a baby is a beautiful occasion, a manifestation of love between two people. Obstetrics are key during pregnancy, so how about giving a presentation about the latest breakthrough in this field? Our free medical template will come in handy.
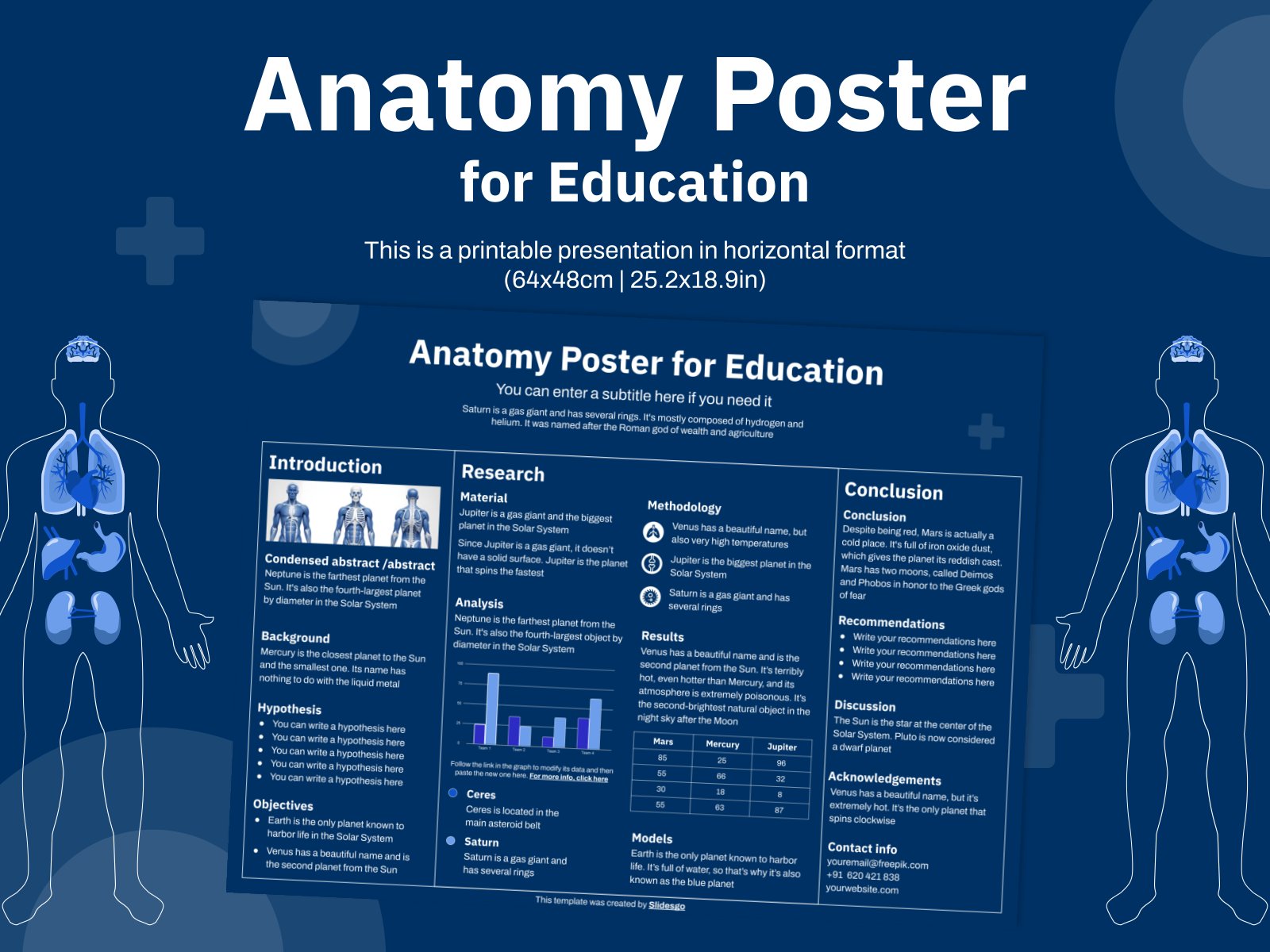
Anatomy Poster for Education
Download the Anatomy Poster for Education presentation for PowerPoint or Google Slides. The education sector constantly demands dynamic and effective ways to present information. This template is created with that very purpose in mind. Offering the best resources, it allows educators or students to efficiently manage their presentations and engage...
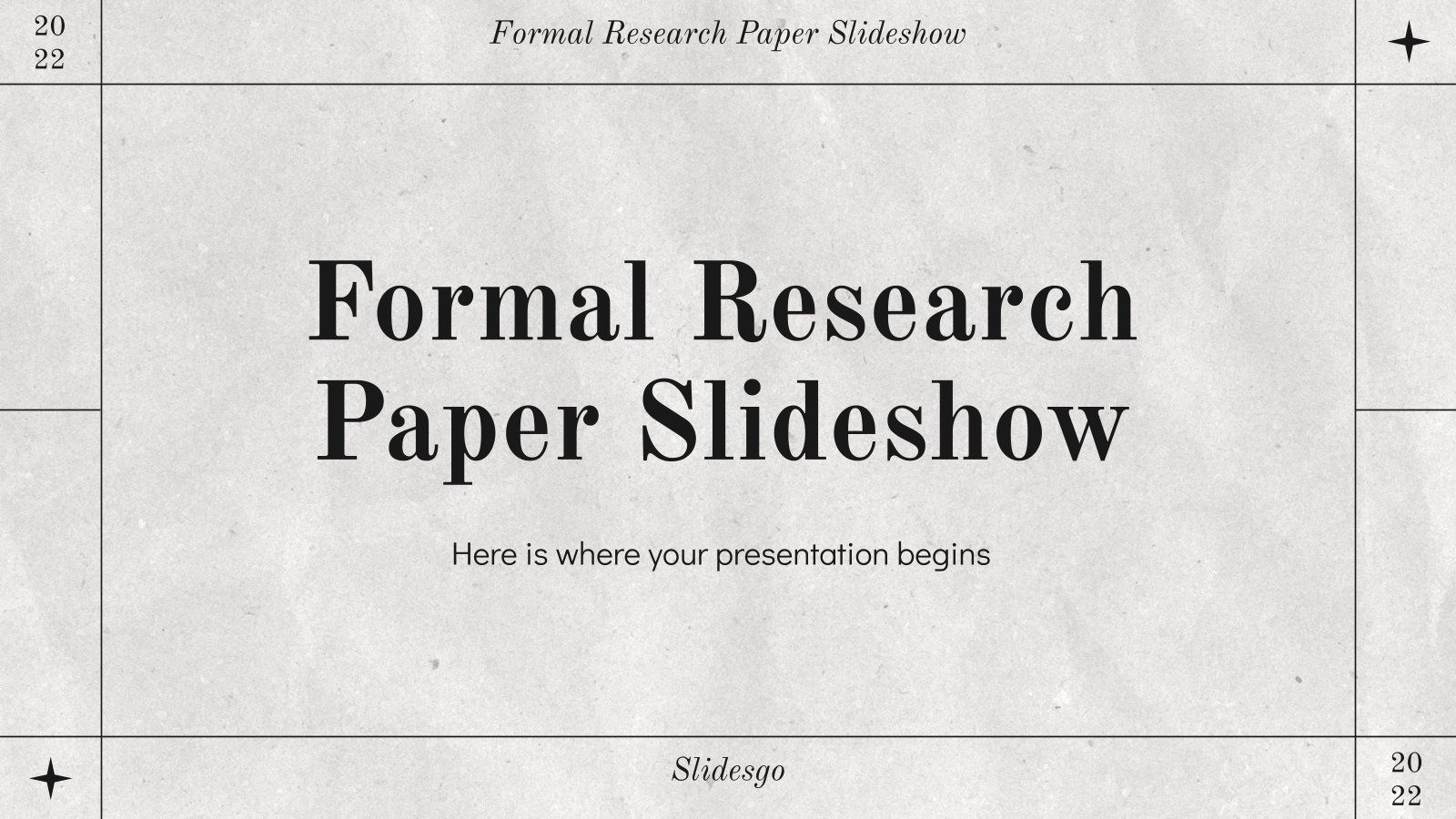
Formal Research Paper Slideshow
Have you seen these slides? They are perfect for presenting your research paper! First of all, because we have included all the necessary sections of this type of work, such as hypothesis, objectives, methodology, analysis and the conclusions of the paper. The second reason is that the formal style will...
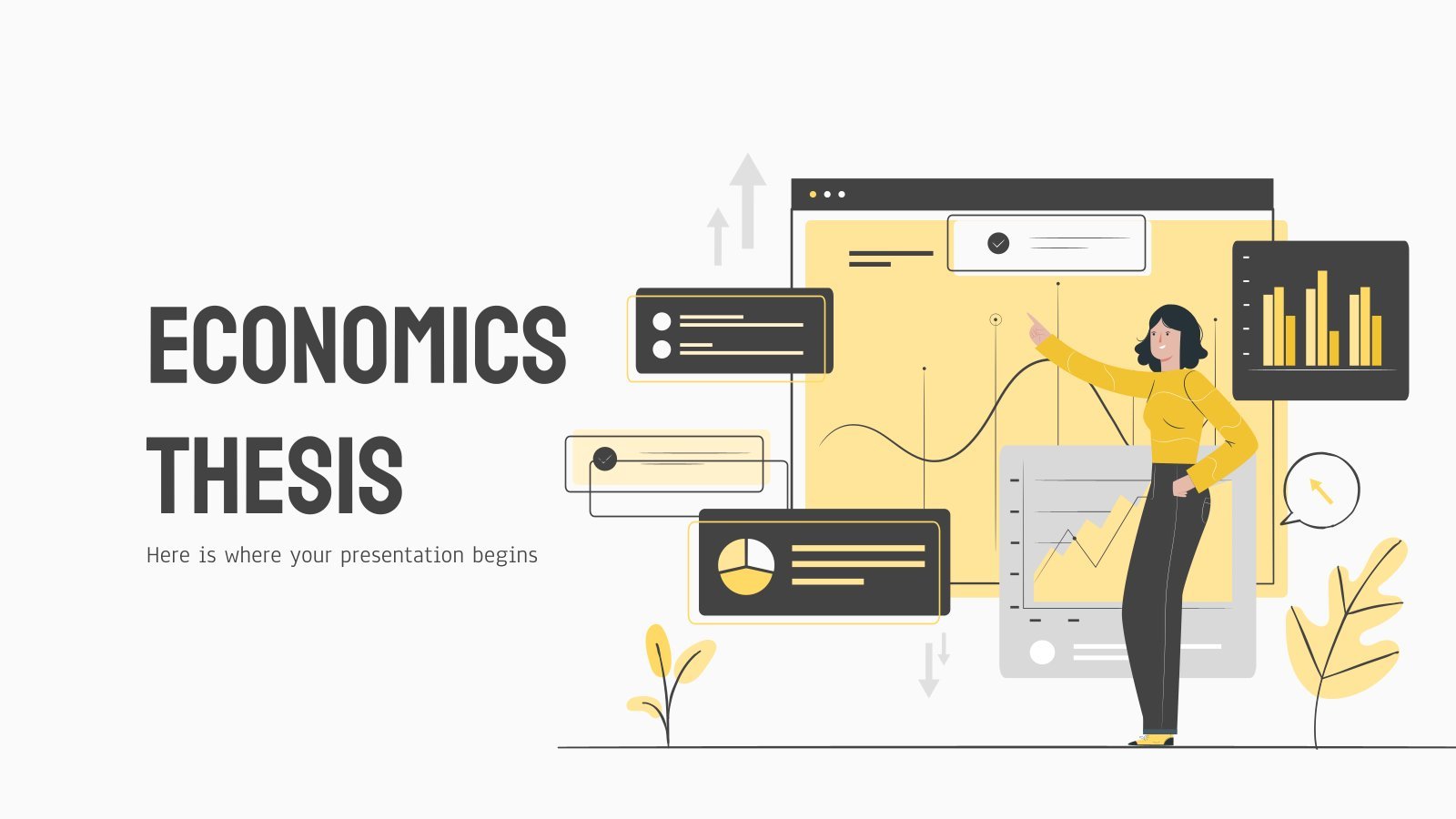
Economics Thesis
If numbers, exchange rates, money and trading are your forte, odds are you’re already working on an economics thesis for your master’s degree. Defending your dissertation is the last step and the most difficult one, but Slidesgo can help you. Here’s our new free presentation template with a focus on...
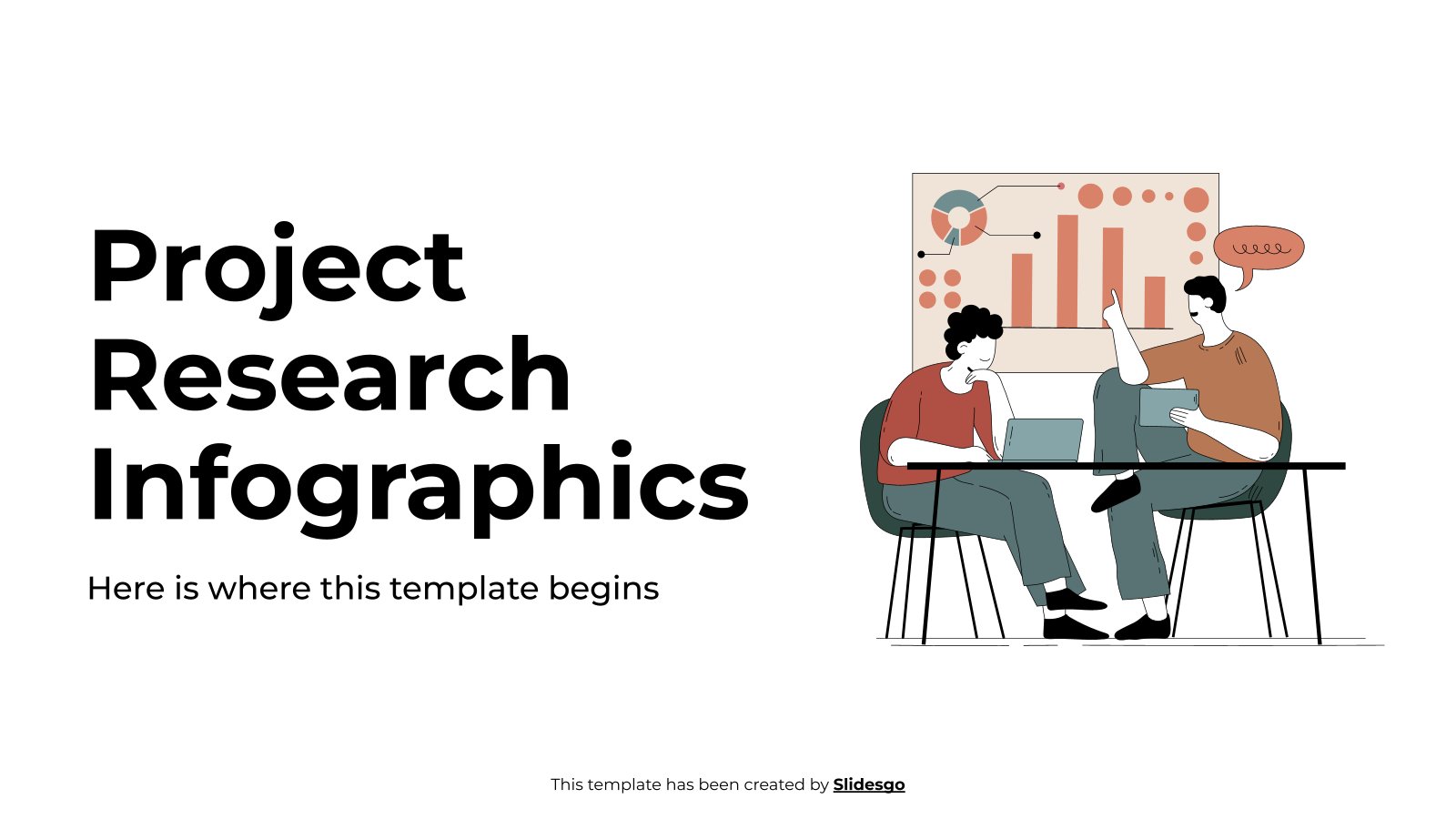
Project Research Infographics
Download the "Project Research Infographics" template for PowerPoint or Google Slides and discover the power of infographics. An infographic resource gives you the ability to showcase your content in a more visual way, which will make it easier for your audience to understand your topic. Slidesgo infographics like this set...
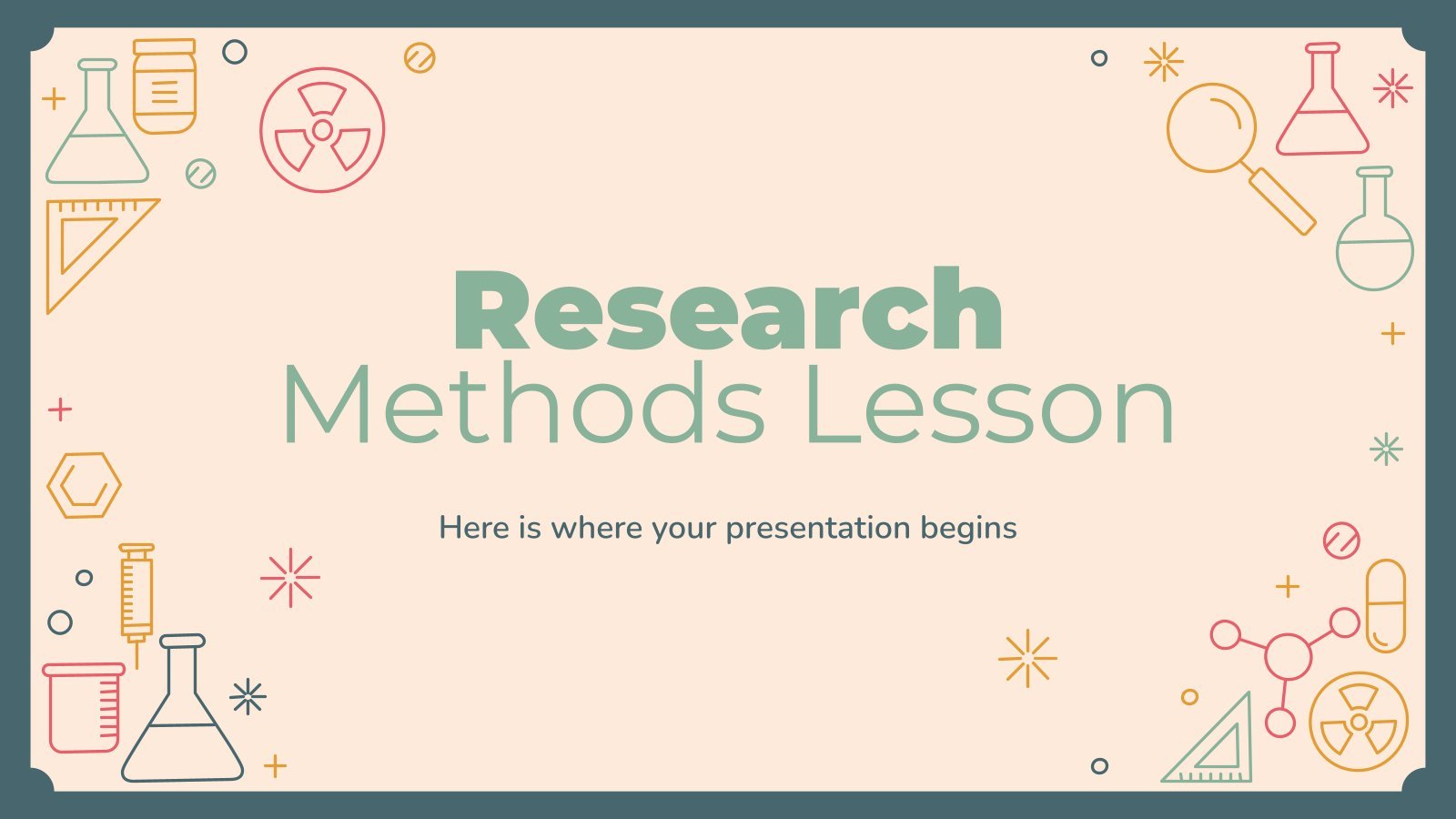
Research Methods Lesson
If you deal with Science, it’s important to learn more about research methods. Teach your students about them with this presentation full of illustrations and drawings related to labs. Use graphs, maps, tables and overview diagrams to support your lecture in a visual way!

Elegant Black & White Thesis Defense
Present your research findings with grace and assertiveness through this template. Available for Google Slides and PowerPoint, this design set offers minimalistic charm with its simple, gray scale elegance. The template not only provides a polished platform to showcase your thesis but also ensures seamless and efficient delivery of your...
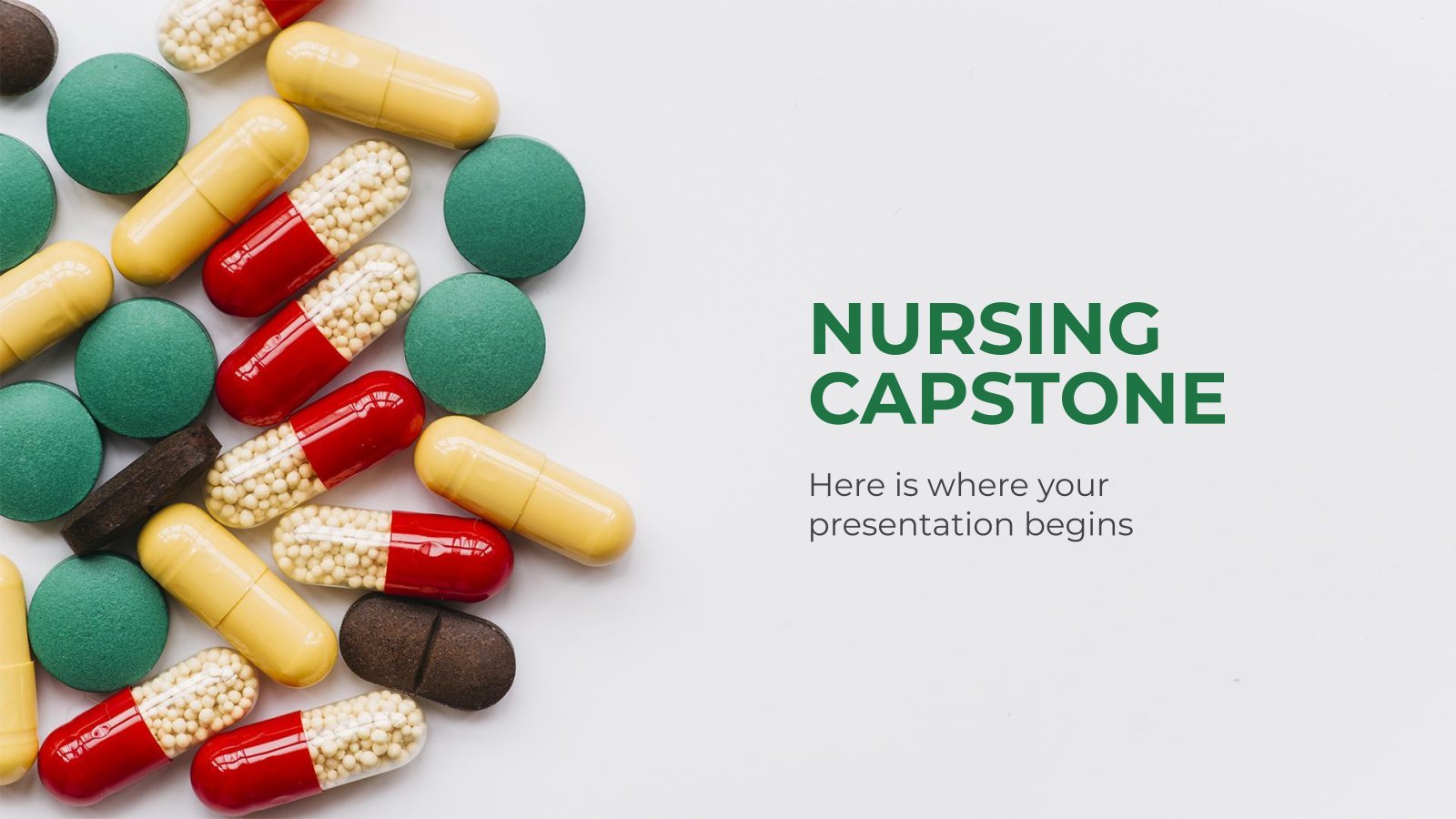
Nursing Capstone
In medical contexts, a capstone is often the final course in a nursing degree, a project of vital importance. It’s very demanding, so if you need help with the presentation, use this free professional template. Leave the design to us and focus on your data!
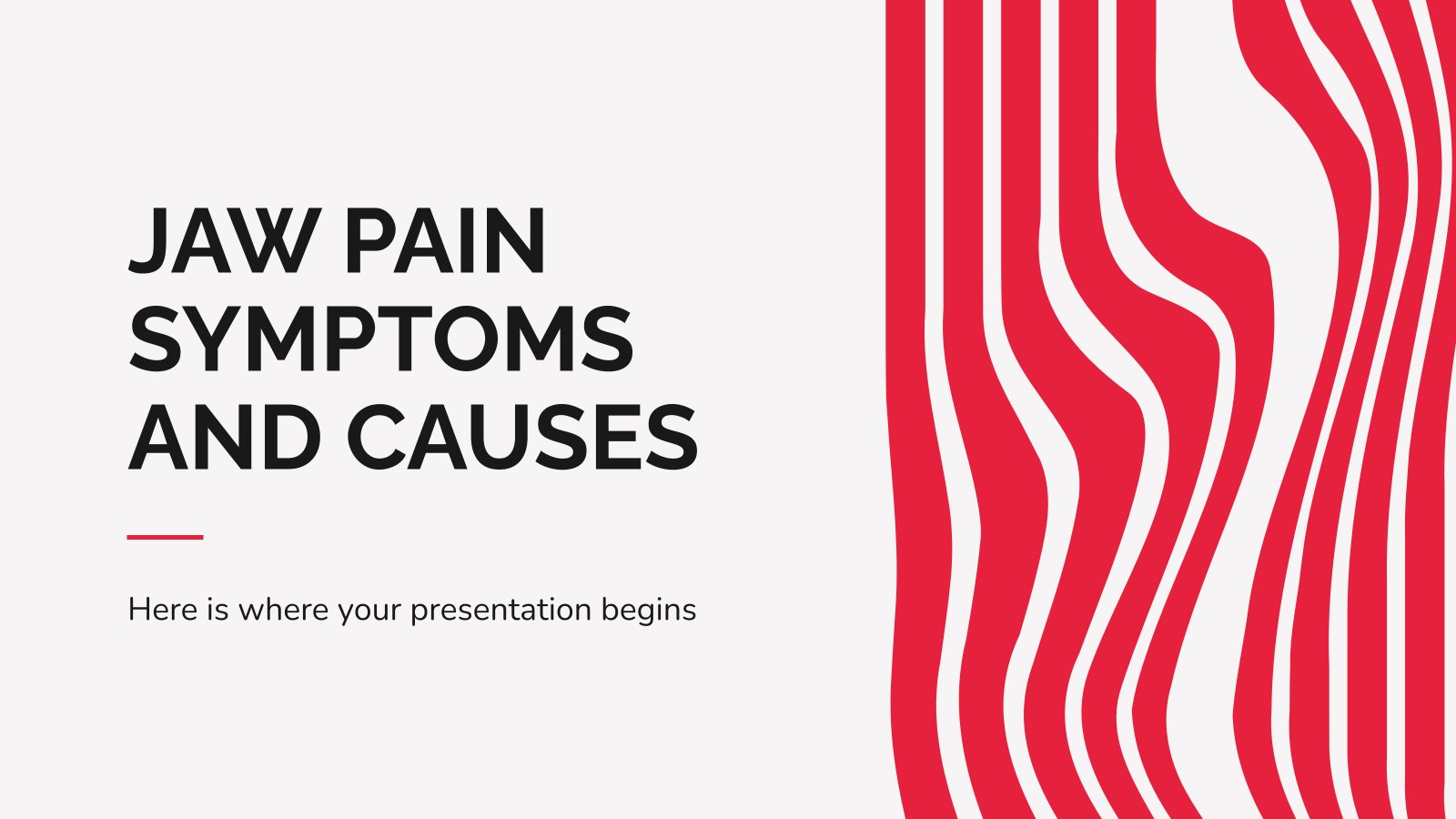
Jaw Pain Symptoms and Causes
Download the Jaw Pain Symptoms and Causes presentation for PowerPoint or Google Slides. Taking care of yourself and of those around you is key! By learning about various illnesses and how they are spread, people can get a better understanding of them and make informed decisions about eating, exercise, and...
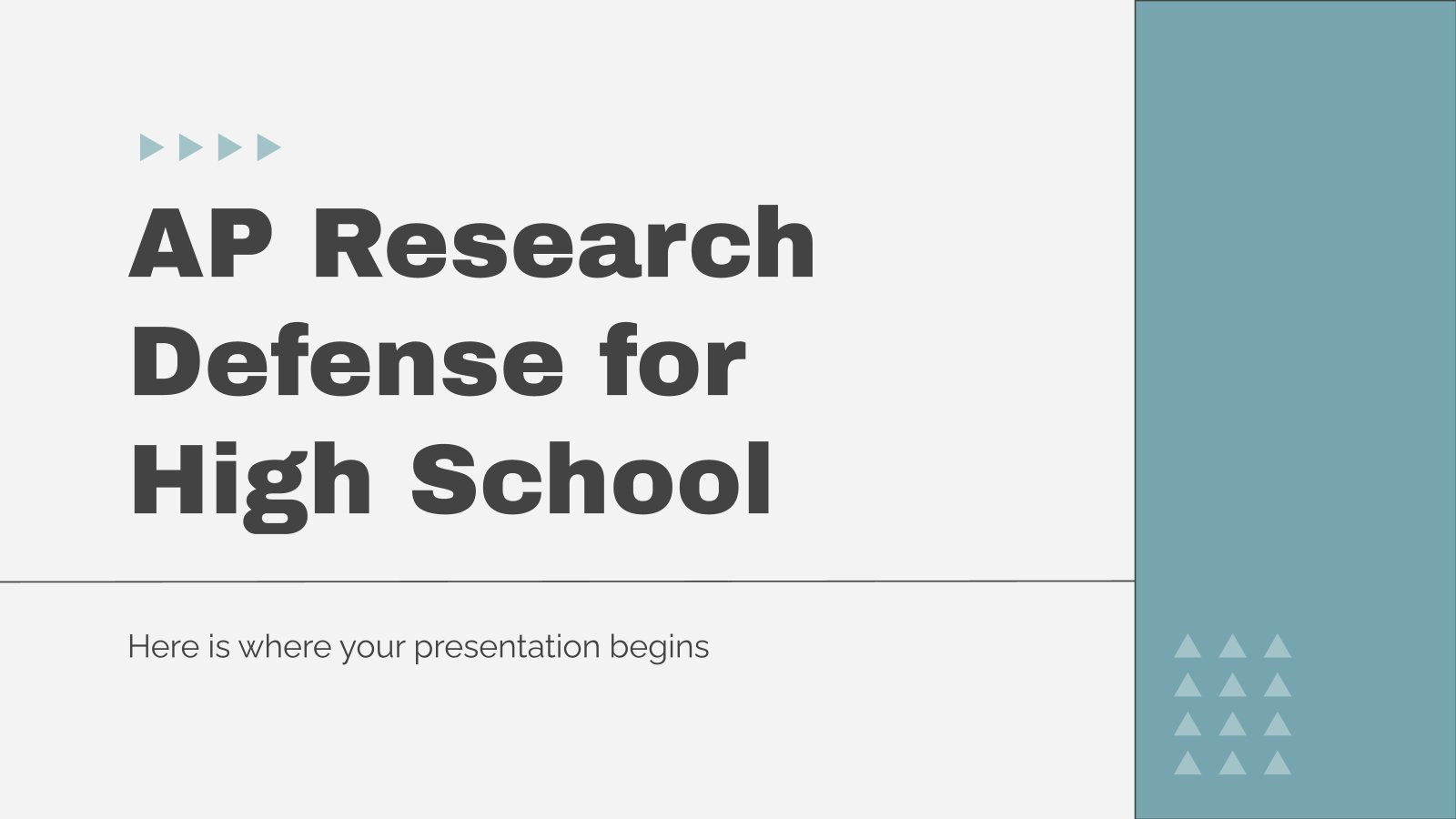
AP Research Defense for High School
AP, or Advanced Placement, is a North American educational program that offers a rigorous course designed to challenge and prepare high school students for their future careers and academic pursuits. It requires students to conduct independent research, write a lengthy academic paper, and present their findings to a panel of...
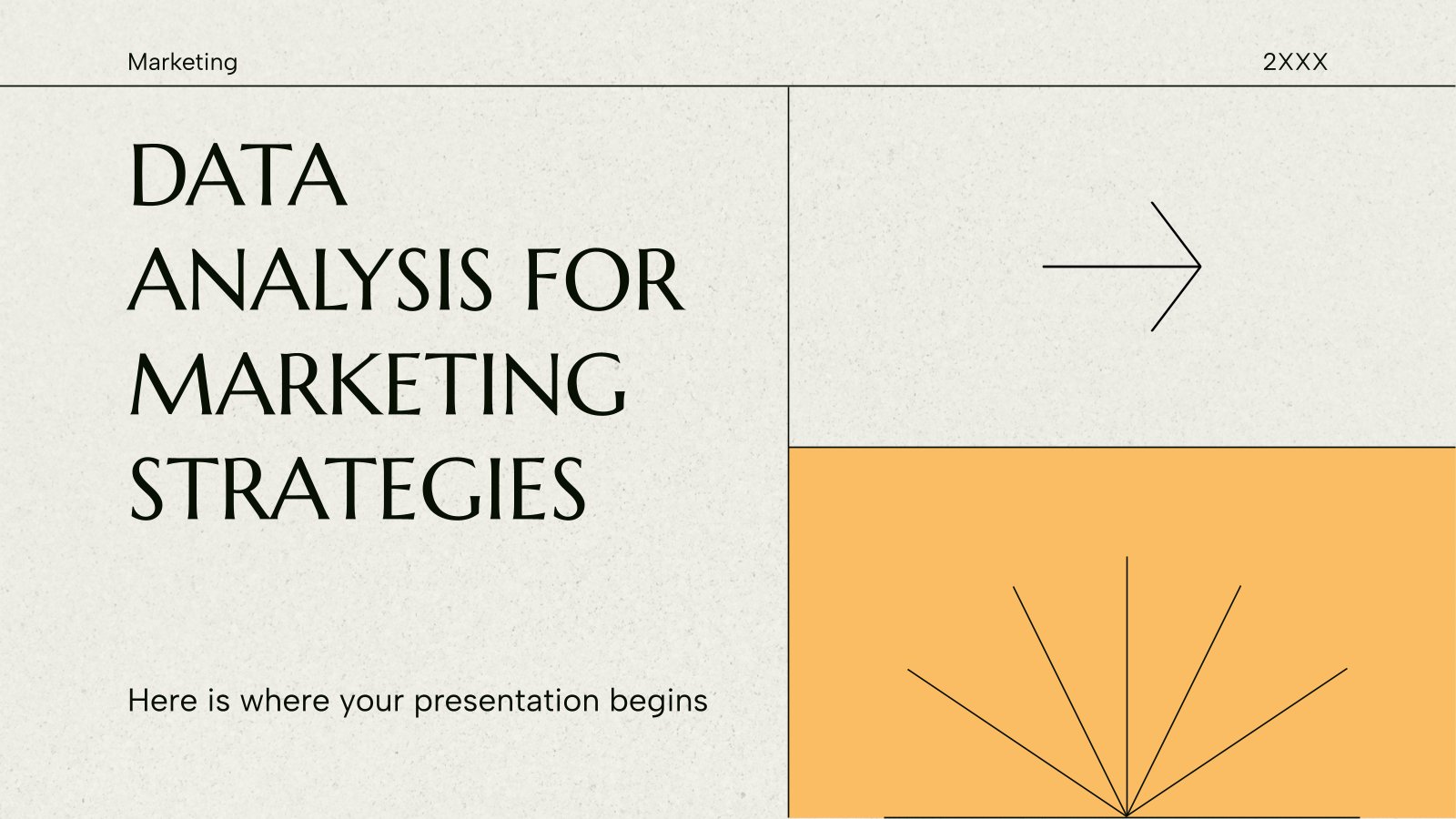
Data Analysis for Marketing Strategies
With the amount of data available through various digital platforms, it's easier than ever to determine the trends and preferences of your target audience. By collecting and analyzing data, marketers can create highly personalized campaigns that align with the exact needs and wants of their customers. If you're trying to...
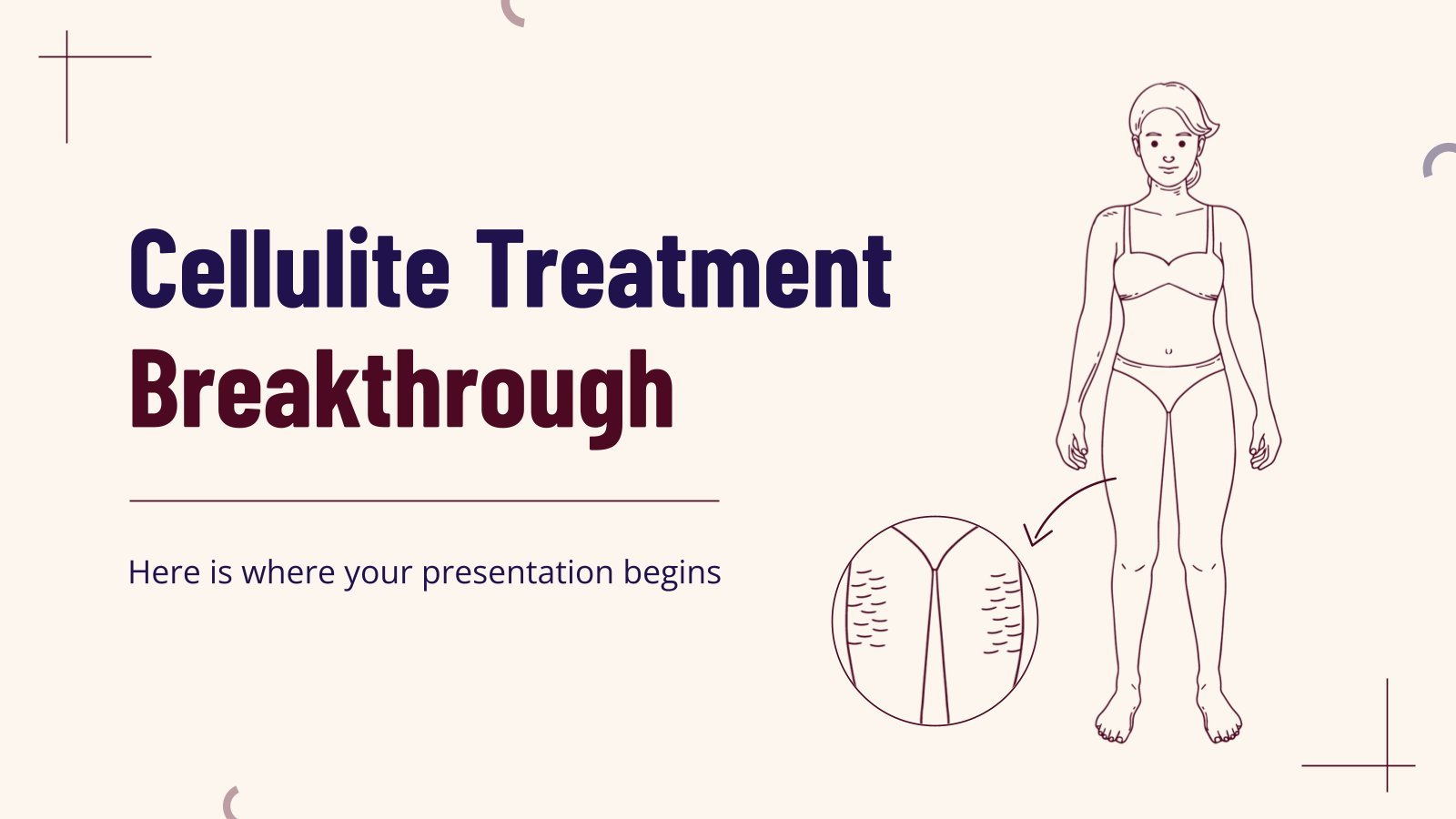
Cellulite Treatment Breakthrough
Download the Cellulite Treatment Breakthrough presentation for PowerPoint or Google Slides.Treating diseases involves a lot of prior research and clinical trials. But whenever there’s a new discovery, a revolutionary finding that opens the door to new treatments, vaccines or ways to prevent illnesses, it’s great news. Should there be a...
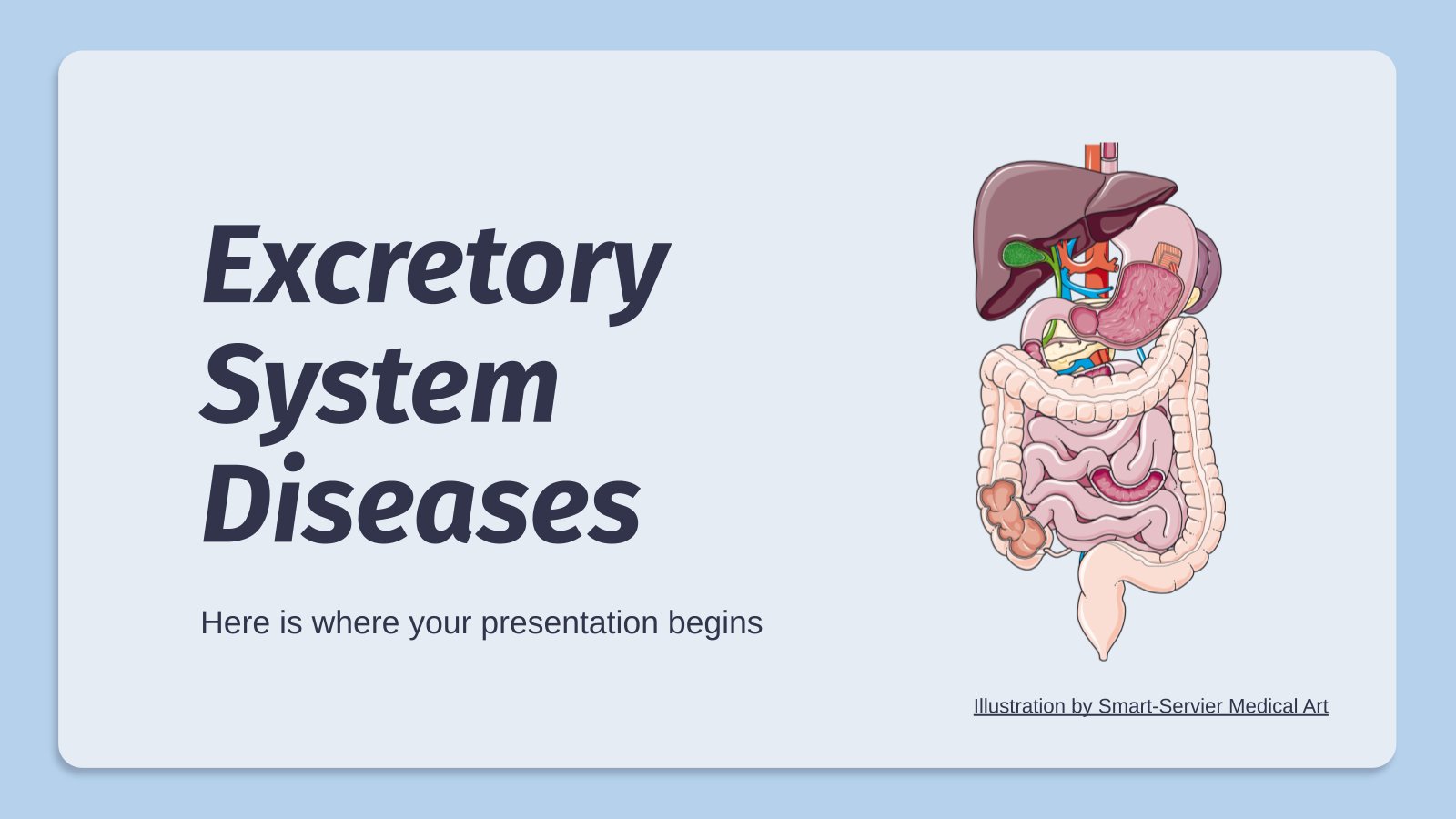
Excretory System Diseases
Download the "Excretory System Diseases" presentation for PowerPoint or Google Slides. Taking care of yourself and of those around you is key! By learning about various illnesses and how they are spread, people can get a better understanding of them and make informed decisions about eating, exercise, and seeking medical...
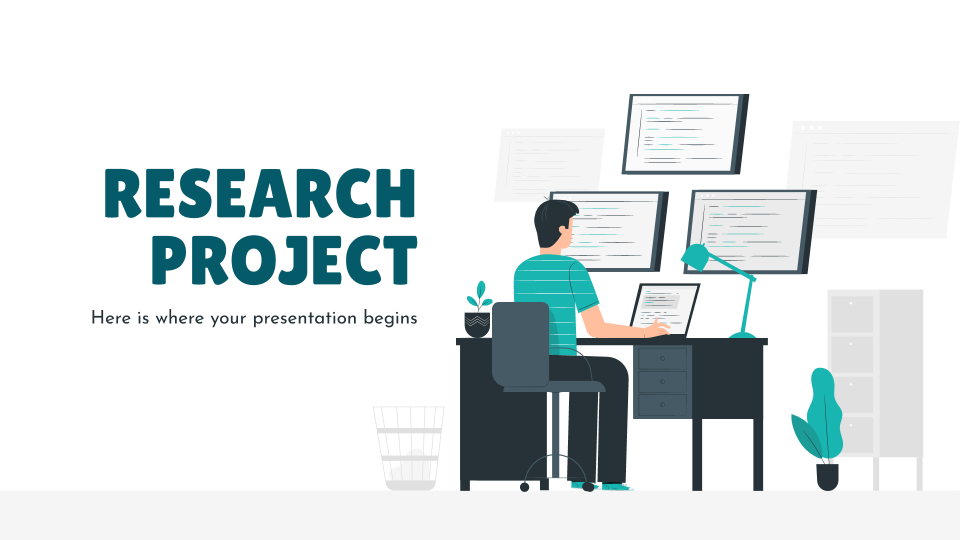
Research Project Proposal
Before embarking yourself on a new project, especially if it’s about research, you need to set out a proposal to explain its viability. Here at Slidesgo we’re offering this theme that you can actually use for any kind of project, regardless of the topic.
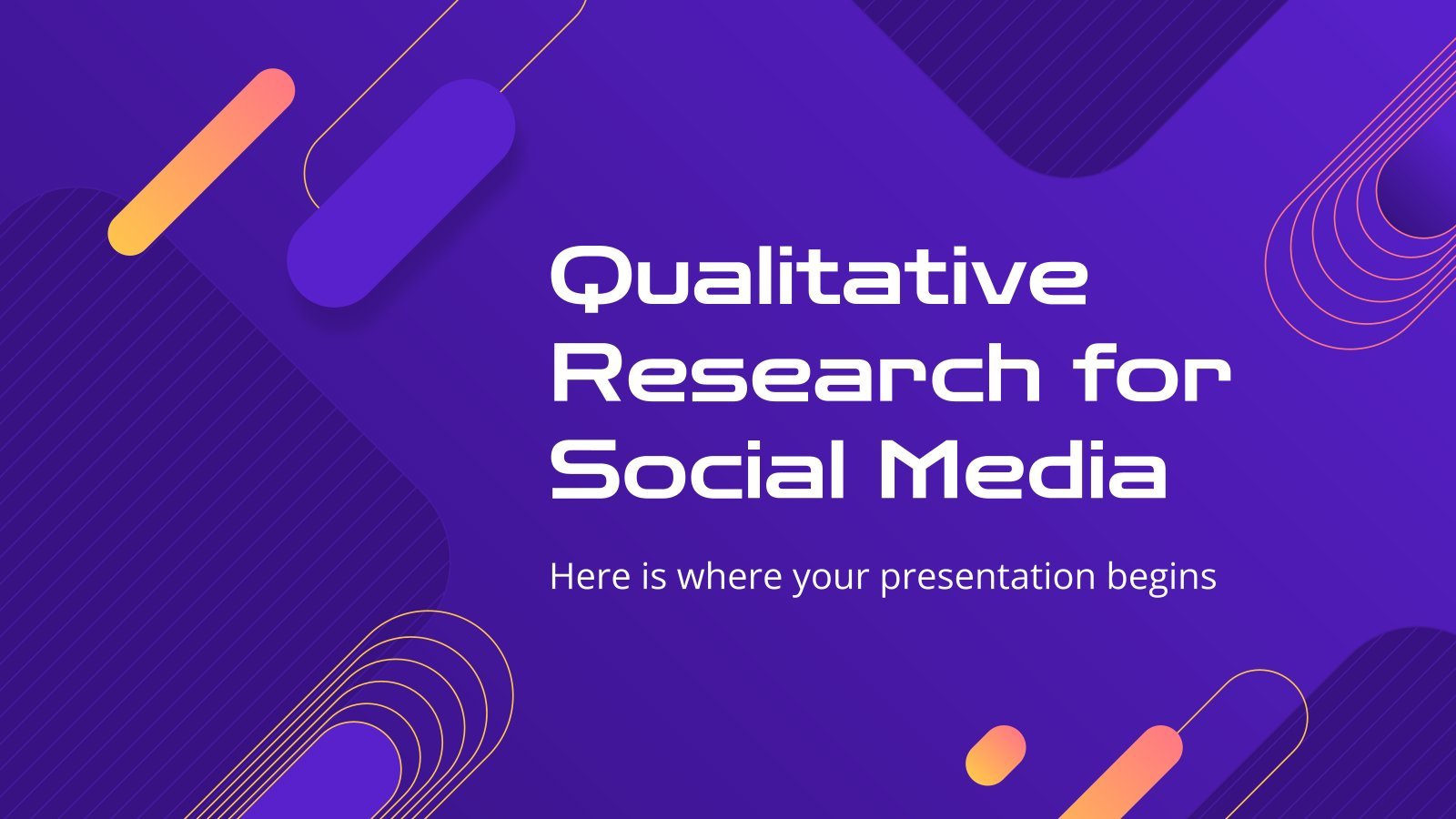
Qualitative Research for Social Media
Download the Qualitative Research for Social Media presentation for PowerPoint or Google Slides. How do you use social media platforms to achieve your business goals? If you need a thorough and professional tool to plan and keep track of your social media strategy, this fully customizable template is your ultimate...
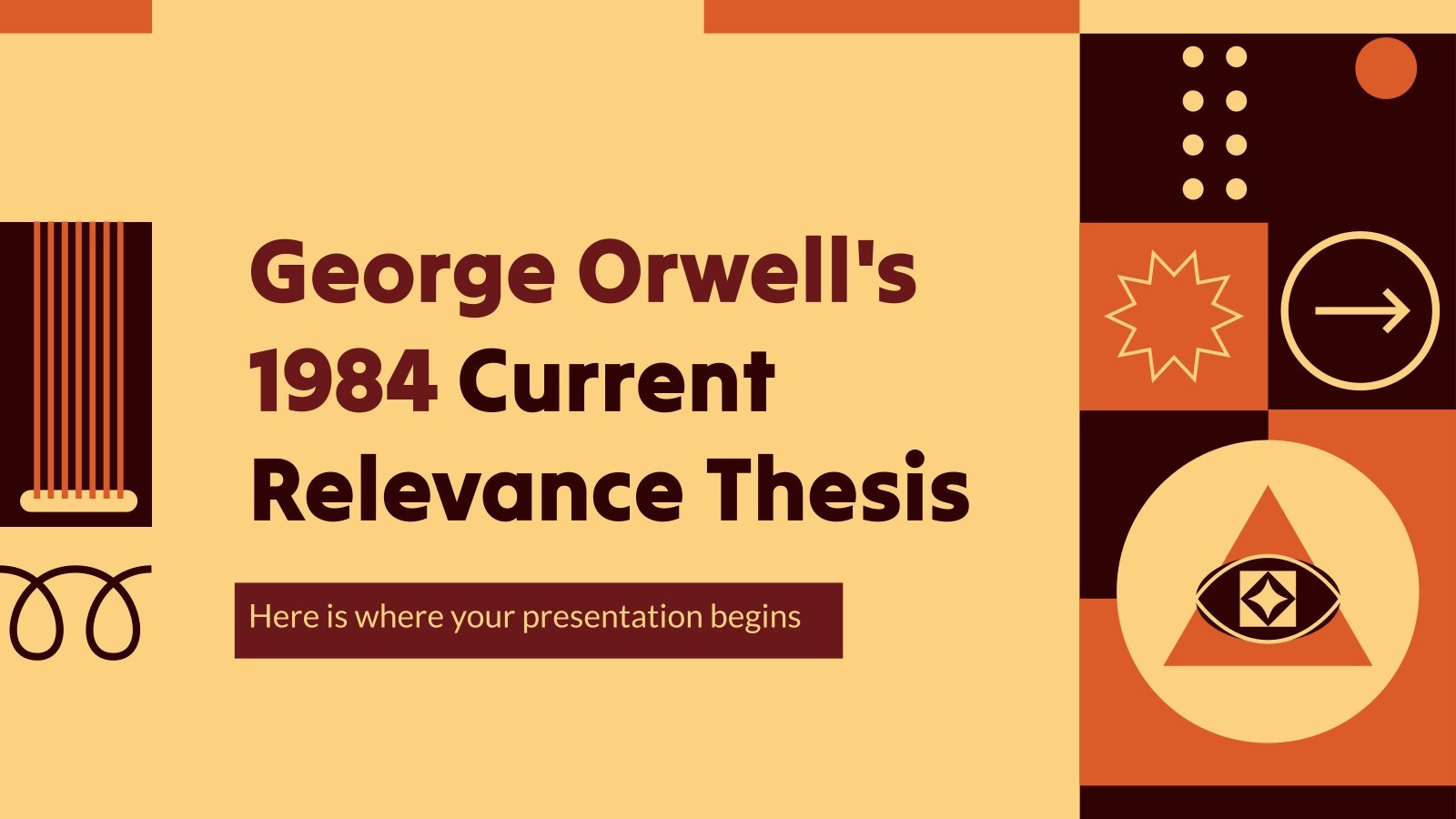
George Orwell's 1984 Current Relevance Thesis
Download the George Orwell's 1984 Current Relevance Thesis presentation for PowerPoint or Google Slides. Congratulations, you have finally finished your research and made it to the end of your thesis! But now comes the big moment: the thesis defense. You want to make sure you showcase your research in the...
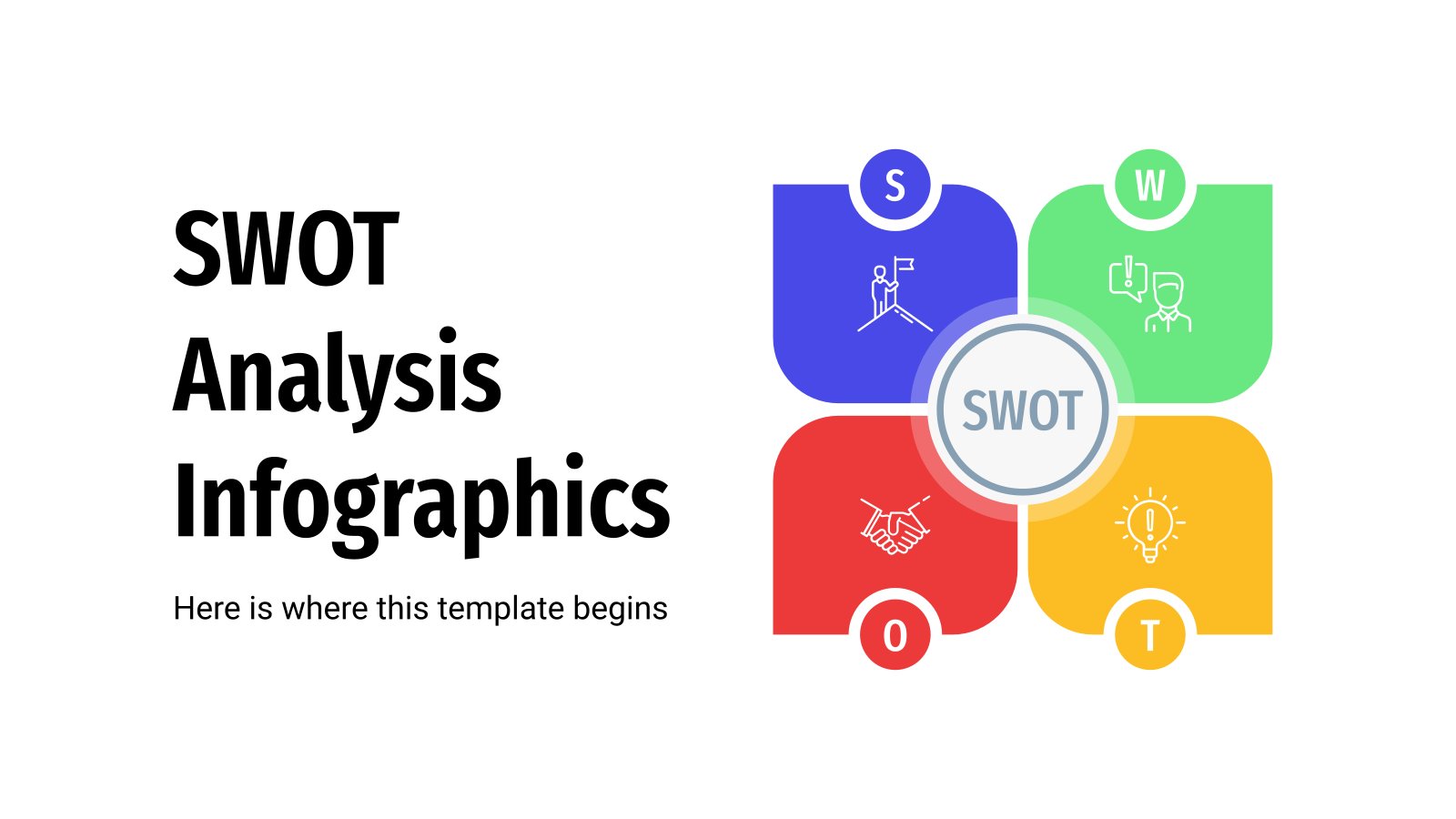
SWOT Analysis Infographics
Discover the strengths, weaknesses, opportunities and threats of your own company performing a SWOT analysis. Use this basic strategic planning to evaluate your position with these new infographics created by Slidesgo.
- Page 1 of 91
Great presentations, faster
Slidesgo for Google Slides :
The easy way to wow
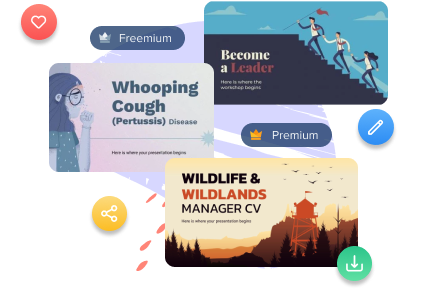
Register for free and start editing online

What’s Included: Research Paper Template
If you’re preparing to write an academic research paper, our free research paper template is the perfect starting point. In the template, we cover every section step by step, with clear, straightforward explanations and examples .
The template’s structure is based on the tried and trusted best-practice format for formal academic research papers. The template structure reflects the overall research process, ensuring your paper will have a smooth, logical flow from chapter to chapter.
The research paper template covers the following core sections:
- The title page/cover page
- Abstract (sometimes also called the executive summary)
- Section 1: Introduction
- Section 2: Literature review
- Section 3: Methodology
- Section 4: Findings /results
- Section 5: Discussion
- Section 6: Conclusion
- Reference list
Each section is explained in plain, straightforward language , followed by an overview of the key elements that you need to cover within each section. We’ve also included links to free resources to help you understand how to write each section.
The cleanly formatted Google Doc can be downloaded as a fully editable MS Word Document (DOCX format), so you can use it as-is or convert it to LaTeX.
FAQs: Research Paper Template
What format is the template (doc, pdf, ppt, etc.).
The research paper template is provided as a Google Doc. You can download it in MS Word format or make a copy to your Google Drive. You’re also welcome to convert it to whatever format works best for you, such as LaTeX or PDF.
What types of research papers can this template be used for?
The template follows the standard best-practice structure for formal academic research papers, so it is suitable for the vast majority of degrees, particularly those within the sciences.
Some universities may have some additional requirements, but these are typically minor, with the core structure remaining the same. Therefore, it’s always a good idea to double-check your university’s requirements before you finalise your structure.
Is this template for an undergrad, Masters or PhD-level research paper?
This template can be used for a research paper at any level of study. It may be slight overkill for an undergraduate-level study, but it certainly won’t be missing anything.
How long should my research paper be?
This depends entirely on your university’s specific requirements, so it’s best to check with them. We include generic word count ranges for each section within the template, but these are purely indicative.
What about the research proposal?
If you’re still working on your research proposal, we’ve got a template for that here .
We’ve also got loads of proposal-related guides and videos over on the Grad Coach blog .
How do I write a literature review?
We have a wealth of free resources on the Grad Coach Blog that unpack how to write a literature review from scratch. You can check out the literature review section of the blog here.
How do I create a research methodology?
We have a wealth of free resources on the Grad Coach Blog that unpack research methodology, both qualitative and quantitative. You can check out the methodology section of the blog here.
Can I share this research paper template with my friends/colleagues?
Yes, you’re welcome to share this template. If you want to post about it on your blog or social media, all we ask is that you reference this page as your source.
Can Grad Coach help me with my research paper?
Within the template, you’ll find plain-language explanations of each section, which should give you a fair amount of guidance. However, you’re also welcome to consider our private coaching services .
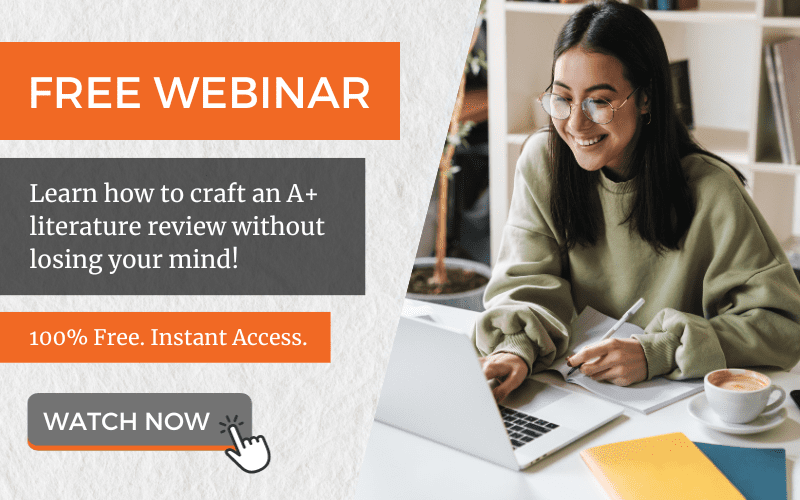
- Affiliate Program

- UNITED STATES
- 台灣 (TAIWAN)
- TÜRKIYE (TURKEY)
- Academic Editing Services
- - Research Paper
- - Journal Manuscript
- - Dissertation
- - College & University Assignments
- Admissions Editing Services
- - Application Essay
- - Personal Statement
- - Recommendation Letter
- - Cover Letter
- - CV/Resume
- Business Editing Services
- - Business Documents
- - Report & Brochure
- - Website & Blog
- Writer Editing Services
- - Script & Screenplay
- Our Editors
- Client Reviews
- Editing & Proofreading Prices
- Wordvice Points
- Partner Discount
- Plagiarism Checker
- APA Citation Generator
- MLA Citation Generator
- Chicago Citation Generator
- Vancouver Citation Generator
- - APA Style
- - MLA Style
- - Chicago Style
- - Vancouver Style
- Writing & Editing Guide
- Academic Resources
- Admissions Resources
How to Write the Results/Findings Section in Research
What is the research paper Results section and what does it do?
The Results section of a scientific research paper represents the core findings of a study derived from the methods applied to gather and analyze information. It presents these findings in a logical sequence without bias or interpretation from the author, setting up the reader for later interpretation and evaluation in the Discussion section. A major purpose of the Results section is to break down the data into sentences that show its significance to the research question(s).
The Results section appears third in the section sequence in most scientific papers. It follows the presentation of the Methods and Materials and is presented before the Discussion section —although the Results and Discussion are presented together in many journals. This section answers the basic question “What did you find in your research?”
What is included in the Results section?
The Results section should include the findings of your study and ONLY the findings of your study. The findings include:
- Data presented in tables, charts, graphs, and other figures (may be placed into the text or on separate pages at the end of the manuscript)
- A contextual analysis of this data explaining its meaning in sentence form
- All data that corresponds to the central research question(s)
- All secondary findings (secondary outcomes, subgroup analyses, etc.)
If the scope of the study is broad, or if you studied a variety of variables, or if the methodology used yields a wide range of different results, the author should present only those results that are most relevant to the research question stated in the Introduction section .
As a general rule, any information that does not present the direct findings or outcome of the study should be left out of this section. Unless the journal requests that authors combine the Results and Discussion sections, explanations and interpretations should be omitted from the Results.
How are the results organized?
The best way to organize your Results section is “logically.” One logical and clear method of organizing research results is to provide them alongside the research questions—within each research question, present the type of data that addresses that research question.
Let’s look at an example. Your research question is based on a survey among patients who were treated at a hospital and received postoperative care. Let’s say your first research question is:
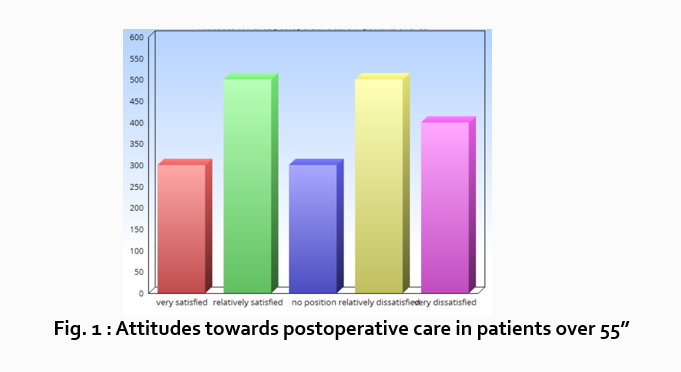
“What do hospital patients over age 55 think about postoperative care?”
This can actually be represented as a heading within your Results section, though it might be presented as a statement rather than a question:
Attitudes towards postoperative care in patients over the age of 55
Now present the results that address this specific research question first. In this case, perhaps a table illustrating data from a survey. Likert items can be included in this example. Tables can also present standard deviations, probabilities, correlation matrices, etc.
Following this, present a content analysis, in words, of one end of the spectrum of the survey or data table. In our example case, start with the POSITIVE survey responses regarding postoperative care, using descriptive phrases. For example:
“Sixty-five percent of patients over 55 responded positively to the question “ Are you satisfied with your hospital’s postoperative care ?” (Fig. 2)
Include other results such as subcategory analyses. The amount of textual description used will depend on how much interpretation of tables and figures is necessary and how many examples the reader needs in order to understand the significance of your research findings.
Next, present a content analysis of another part of the spectrum of the same research question, perhaps the NEGATIVE or NEUTRAL responses to the survey. For instance:
“As Figure 1 shows, 15 out of 60 patients in Group A responded negatively to Question 2.”
After you have assessed the data in one figure and explained it sufficiently, move on to your next research question. For example:
“How does patient satisfaction correspond to in-hospital improvements made to postoperative care?”
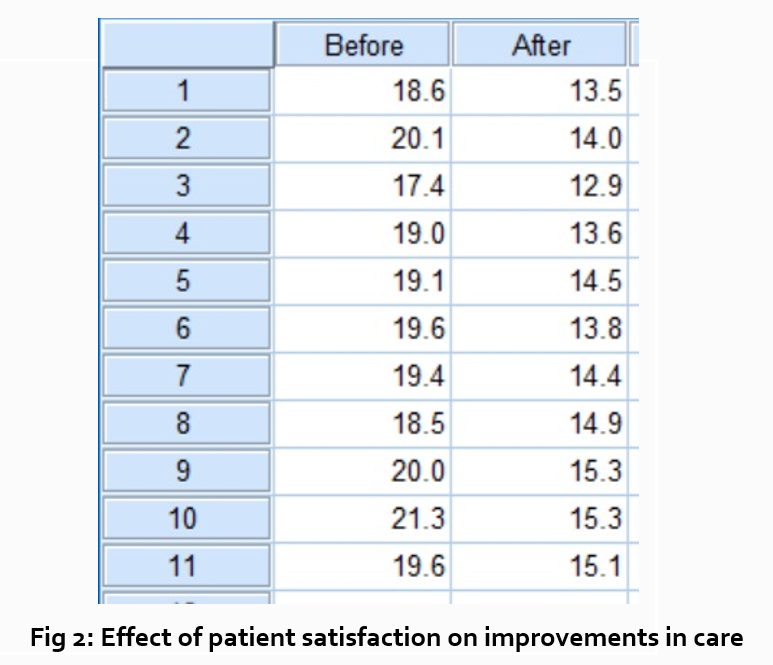
This kind of data may be presented through a figure or set of figures (for instance, a paired T-test table).
Explain the data you present, here in a table, with a concise content analysis:
“The p-value for the comparison between the before and after groups of patients was .03% (Fig. 2), indicating that the greater the dissatisfaction among patients, the more frequent the improvements that were made to postoperative care.”
Let’s examine another example of a Results section from a study on plant tolerance to heavy metal stress . In the Introduction section, the aims of the study are presented as “determining the physiological and morphological responses of Allium cepa L. towards increased cadmium toxicity” and “evaluating its potential to accumulate the metal and its associated environmental consequences.” The Results section presents data showing how these aims are achieved in tables alongside a content analysis, beginning with an overview of the findings:
“Cadmium caused inhibition of root and leave elongation, with increasing effects at higher exposure doses (Fig. 1a-c).”
The figure containing this data is cited in parentheses. Note that this author has combined three graphs into one single figure. Separating the data into separate graphs focusing on specific aspects makes it easier for the reader to assess the findings, and consolidating this information into one figure saves space and makes it easy to locate the most relevant results.
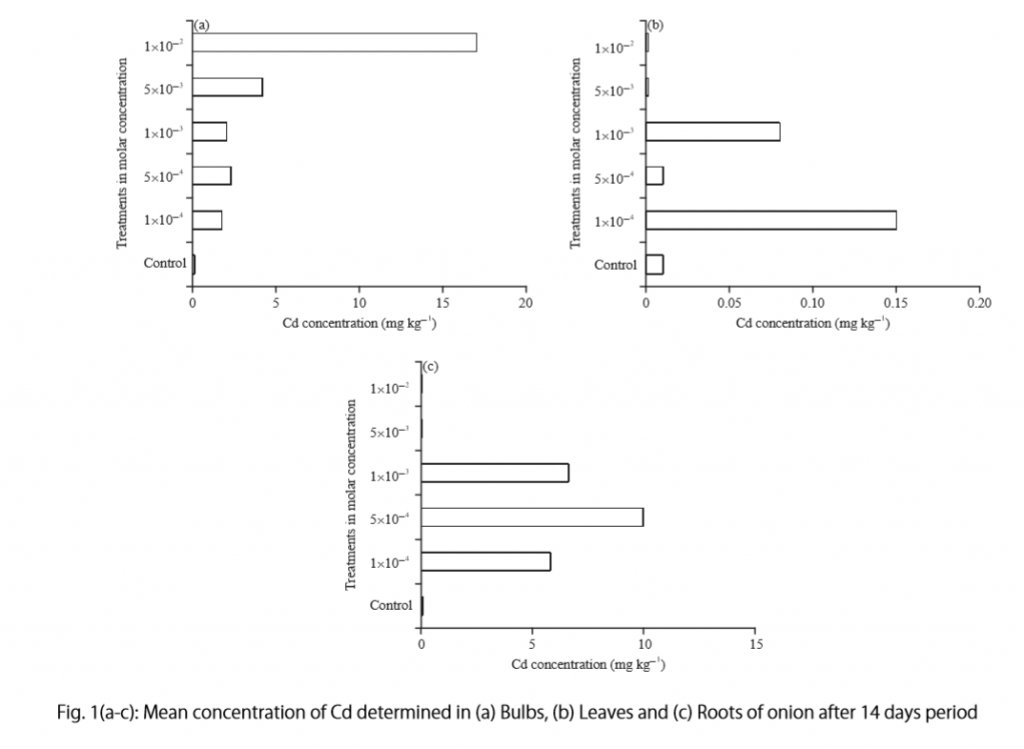
Following this overall summary, the relevant data in the tables is broken down into greater detail in text form in the Results section.
- “Results on the bio-accumulation of cadmium were found to be the highest (17.5 mg kgG1) in the bulb, when the concentration of cadmium in the solution was 1×10G2 M and lowest (0.11 mg kgG1) in the leaves when the concentration was 1×10G3 M.”
Captioning and Referencing Tables and Figures
Tables and figures are central components of your Results section and you need to carefully think about the most effective way to use graphs and tables to present your findings . Therefore, it is crucial to know how to write strong figure captions and to refer to them within the text of the Results section.
The most important advice one can give here as well as throughout the paper is to check the requirements and standards of the journal to which you are submitting your work. Every journal has its own design and layout standards, which you can find in the author instructions on the target journal’s website. Perusing a journal’s published articles will also give you an idea of the proper number, size, and complexity of your figures.
Regardless of which format you use, the figures should be placed in the order they are referenced in the Results section and be as clear and easy to understand as possible. If there are multiple variables being considered (within one or more research questions), it can be a good idea to split these up into separate figures. Subsequently, these can be referenced and analyzed under separate headings and paragraphs in the text.
To create a caption, consider the research question being asked and change it into a phrase. For instance, if one question is “Which color did participants choose?”, the caption might be “Color choice by participant group.” Or in our last research paper example, where the question was “What is the concentration of cadmium in different parts of the onion after 14 days?” the caption reads:
“Fig. 1(a-c): Mean concentration of Cd determined in (a) bulbs, (b) leaves, and (c) roots of onions after a 14-day period.”
Steps for Composing the Results Section
Because each study is unique, there is no one-size-fits-all approach when it comes to designing a strategy for structuring and writing the section of a research paper where findings are presented. The content and layout of this section will be determined by the specific area of research, the design of the study and its particular methodologies, and the guidelines of the target journal and its editors. However, the following steps can be used to compose the results of most scientific research studies and are essential for researchers who are new to preparing a manuscript for publication or who need a reminder of how to construct the Results section.
Step 1 : Consult the guidelines or instructions that the target journal or publisher provides authors and read research papers it has published, especially those with similar topics, methods, or results to your study.
- The guidelines will generally outline specific requirements for the results or findings section, and the published articles will provide sound examples of successful approaches.
- Note length limitations on restrictions on content. For instance, while many journals require the Results and Discussion sections to be separate, others do not—qualitative research papers often include results and interpretations in the same section (“Results and Discussion”).
- Reading the aims and scope in the journal’s “ guide for authors ” section and understanding the interests of its readers will be invaluable in preparing to write the Results section.
Step 2 : Consider your research results in relation to the journal’s requirements and catalogue your results.
- Focus on experimental results and other findings that are especially relevant to your research questions and objectives and include them even if they are unexpected or do not support your ideas and hypotheses.
- Catalogue your findings—use subheadings to streamline and clarify your report. This will help you avoid excessive and peripheral details as you write and also help your reader understand and remember your findings. Create appendices that might interest specialists but prove too long or distracting for other readers.
- Decide how you will structure of your results. You might match the order of the research questions and hypotheses to your results, or you could arrange them according to the order presented in the Methods section. A chronological order or even a hierarchy of importance or meaningful grouping of main themes or categories might prove effective. Consider your audience, evidence, and most importantly, the objectives of your research when choosing a structure for presenting your findings.
Step 3 : Design figures and tables to present and illustrate your data.
- Tables and figures should be numbered according to the order in which they are mentioned in the main text of the paper.
- Information in figures should be relatively self-explanatory (with the aid of captions), and their design should include all definitions and other information necessary for readers to understand the findings without reading all of the text.
- Use tables and figures as a focal point to tell a clear and informative story about your research and avoid repeating information. But remember that while figures clarify and enhance the text, they cannot replace it.
Step 4 : Draft your Results section using the findings and figures you have organized.
- The goal is to communicate this complex information as clearly and precisely as possible; precise and compact phrases and sentences are most effective.
- In the opening paragraph of this section, restate your research questions or aims to focus the reader’s attention to what the results are trying to show. It is also a good idea to summarize key findings at the end of this section to create a logical transition to the interpretation and discussion that follows.
- Try to write in the past tense and the active voice to relay the findings since the research has already been done and the agent is usually clear. This will ensure that your explanations are also clear and logical.
- Make sure that any specialized terminology or abbreviation you have used here has been defined and clarified in the Introduction section .
Step 5 : Review your draft; edit and revise until it reports results exactly as you would like to have them reported to your readers.
- Double-check the accuracy and consistency of all the data, as well as all of the visual elements included.
- Read your draft aloud to catch language errors (grammar, spelling, and mechanics), awkward phrases, and missing transitions.
- Ensure that your results are presented in the best order to focus on objectives and prepare readers for interpretations, valuations, and recommendations in the Discussion section . Look back over the paper’s Introduction and background while anticipating the Discussion and Conclusion sections to ensure that the presentation of your results is consistent and effective.
- Consider seeking additional guidance on your paper. Find additional readers to look over your Results section and see if it can be improved in any way. Peers, professors, or qualified experts can provide valuable insights.
One excellent option is to use a professional English proofreading and editing service such as Wordvice, including our paper editing service . With hundreds of qualified editors from dozens of scientific fields, Wordvice has helped thousands of authors revise their manuscripts and get accepted into their target journals. Read more about the proofreading and editing process before proceeding with getting academic editing services and manuscript editing services for your manuscript.
As the representation of your study’s data output, the Results section presents the core information in your research paper. By writing with clarity and conciseness and by highlighting and explaining the crucial findings of their study, authors increase the impact and effectiveness of their research manuscripts.
For more articles and videos on writing your research manuscript, visit Wordvice’s Resources page.
Wordvice Resources
- How to Write a Research Paper Introduction
- Which Verb Tenses to Use in a Research Paper
- How to Write an Abstract for a Research Paper
- How to Write a Research Paper Title
- Useful Phrases for Academic Writing
- Common Transition Terms in Academic Papers
- Active and Passive Voice in Research Papers
- 100+ Verbs That Will Make Your Research Writing Amazing
- Tips for Paraphrasing in Research Papers
- Get started
Research Report
This template guides students and researchers in writing a research report.
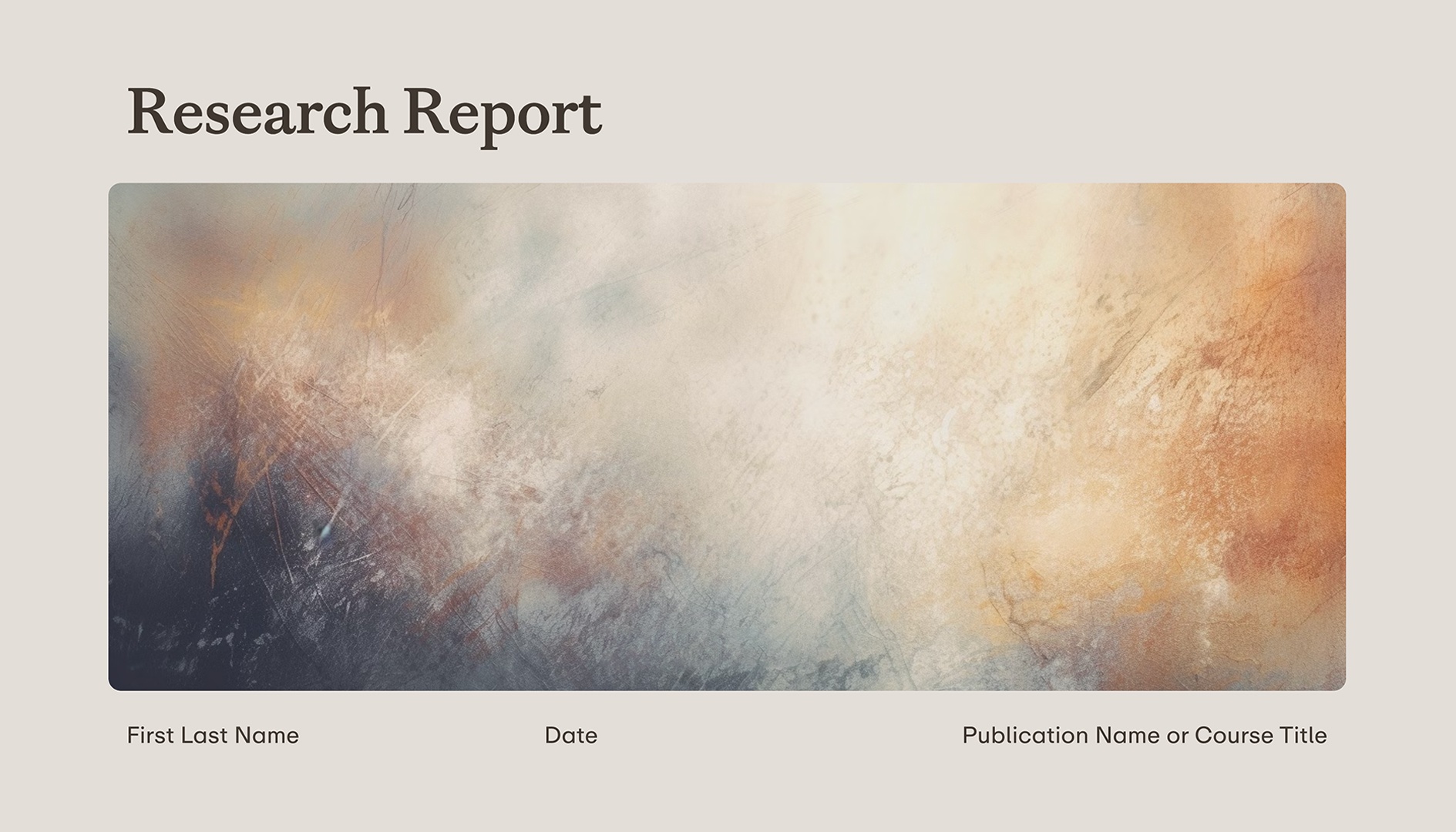
About this template
Everything you need to write a comprehensive research report is in this template. From a Table of Contents page that includes sections sharing guidelines on how to craft an Executive Summary to describing your approach to research and pages for key takeaways and conclusions, this research template helps you communicate in depth your research findings to an audience. Use this template for market research, business research, and more.
Template outline
- Checkmark Cover Page
- Checkmark Table of Contents
- Checkmark Executive Summary
- Checkmark Approach
- Checkmark Subject Matter/Industry Overview
- Checkmark Sample Selection & Analysis
- Checkmark Key Topic or Segmentation Page
- Checkmark Analysis Page
- Checkmark Takeaways & Recommendations
- Checkmark Sources Cited Page
- Checkmark Thank You Page
Tell a powerful story
- Double Sparkle (tome icon) Editable with AI
- Page Portrait (tome icon) Narrative Guidance
- Share (tome icon) Share or link anywhere
- Text (tome icon) Beautiful typography
- Multiple Pages (tome icon) Automatic mobile layout
- Download (tome icon) Downloadable as a PDF
- Double Sparkle (tome icon) AI layout generation
- Reference (tome icon) AI reference sourcing
- Add Person (tome icon) Real-time collaboration
More templates
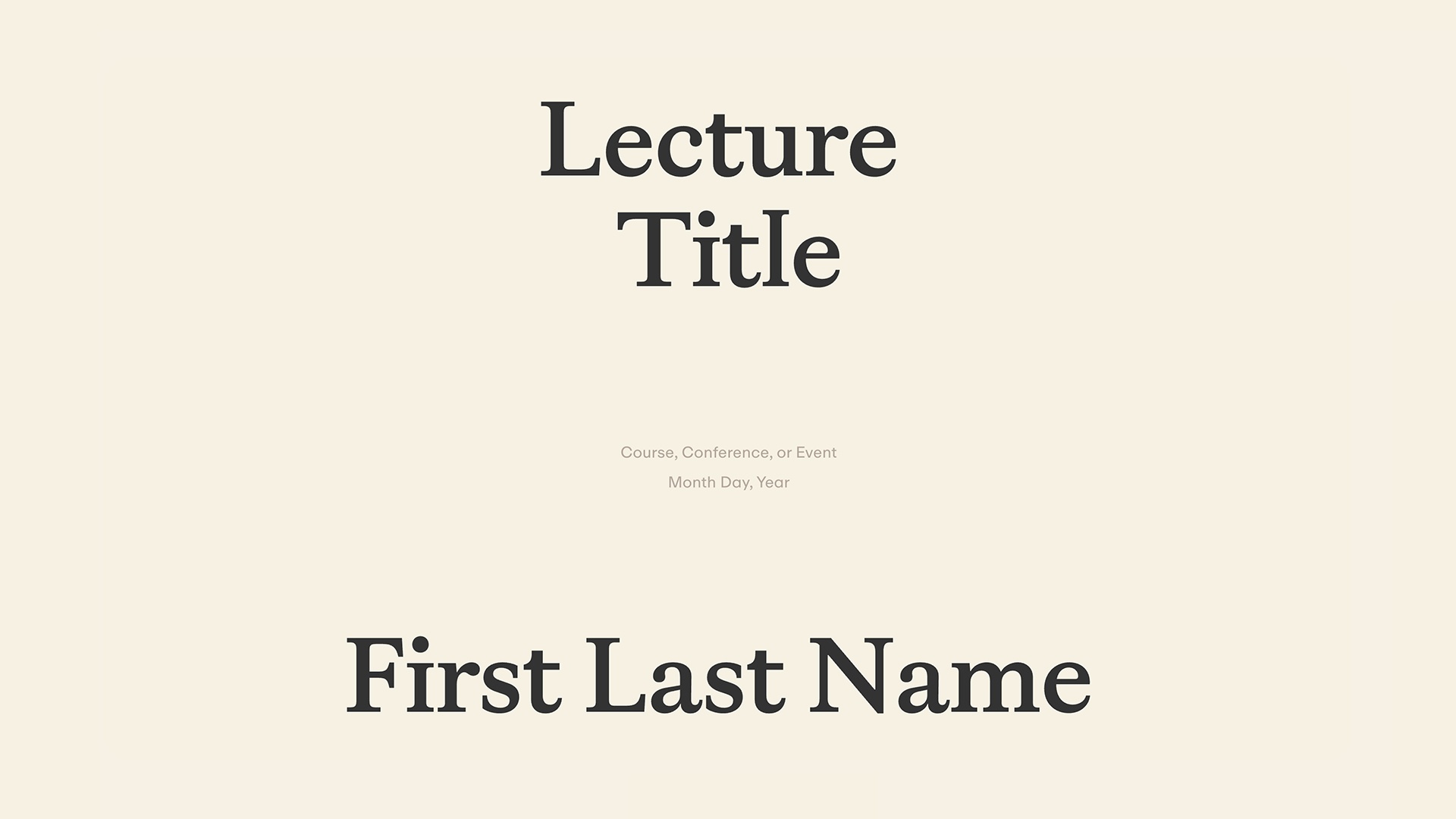
One Page Website
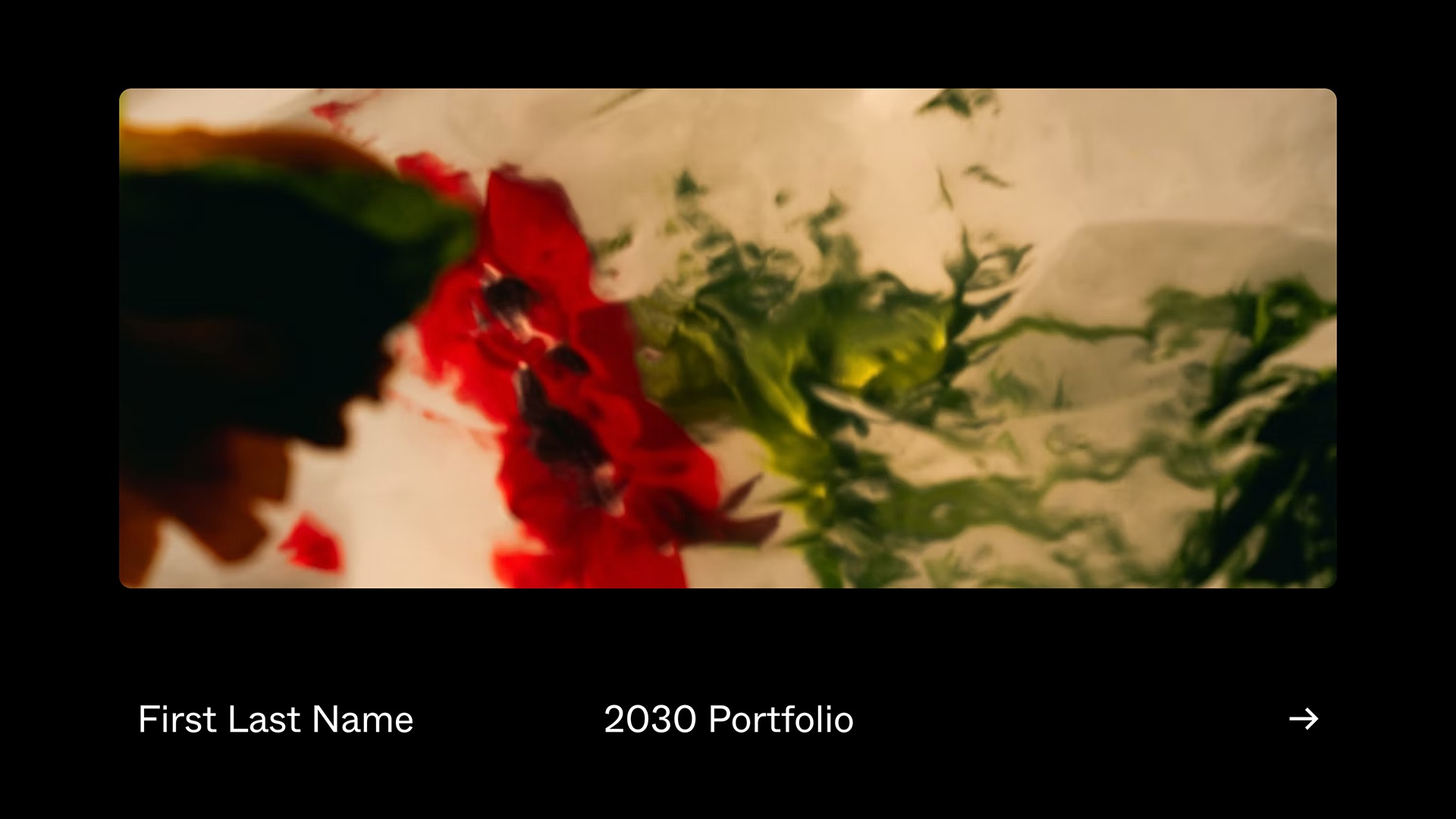
Work Portfolio
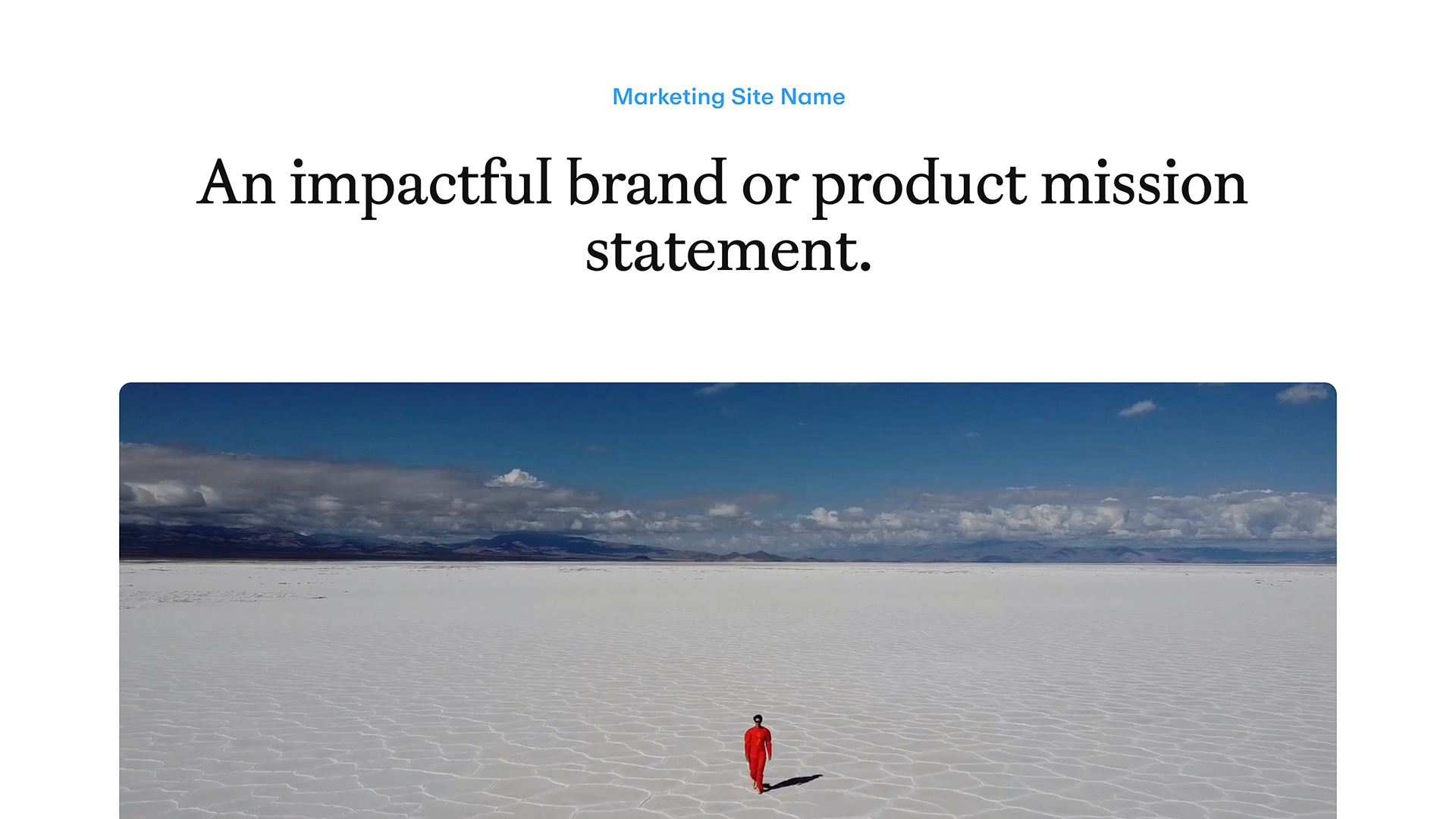
Marketing Site
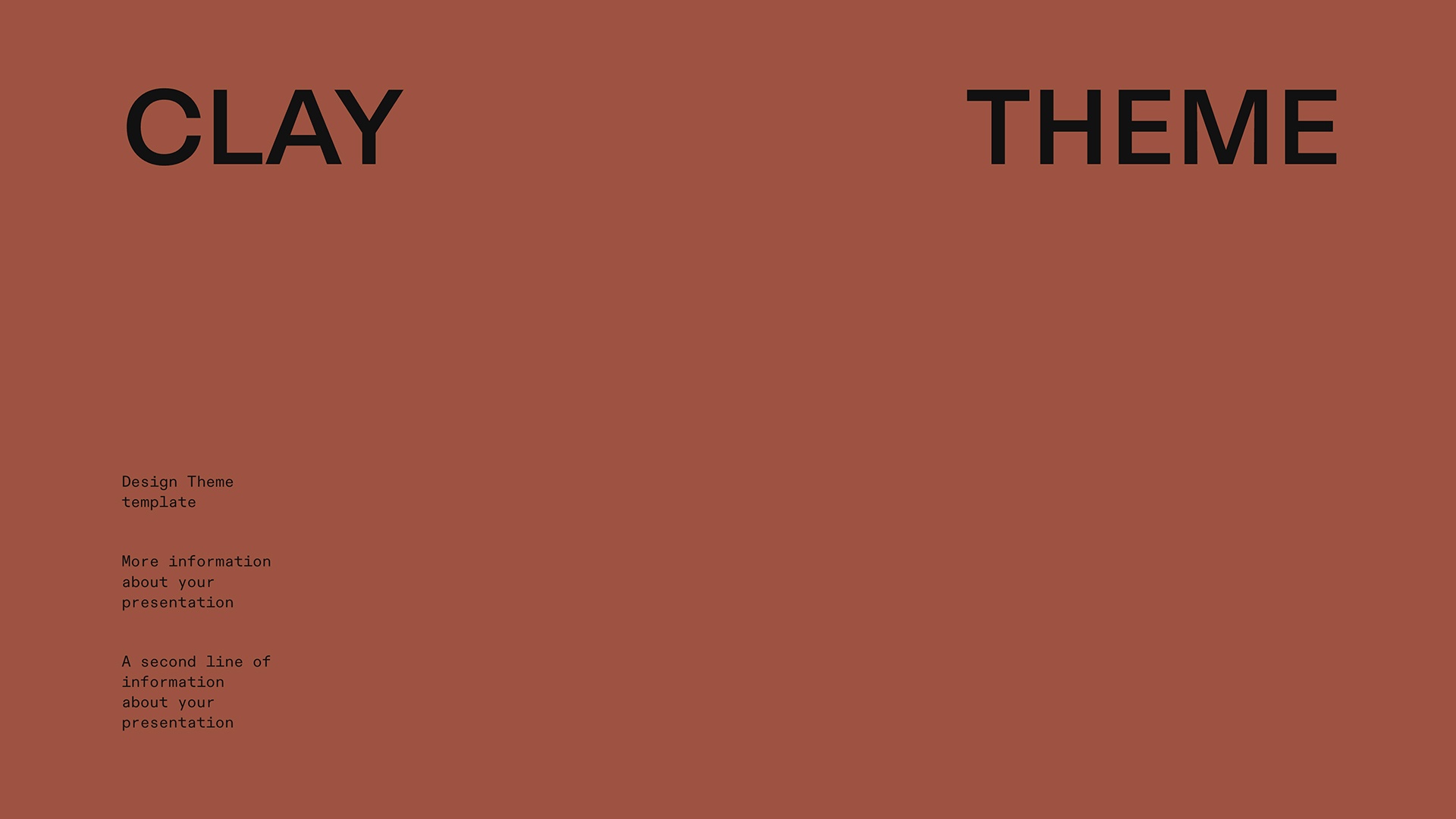
Product Design Review
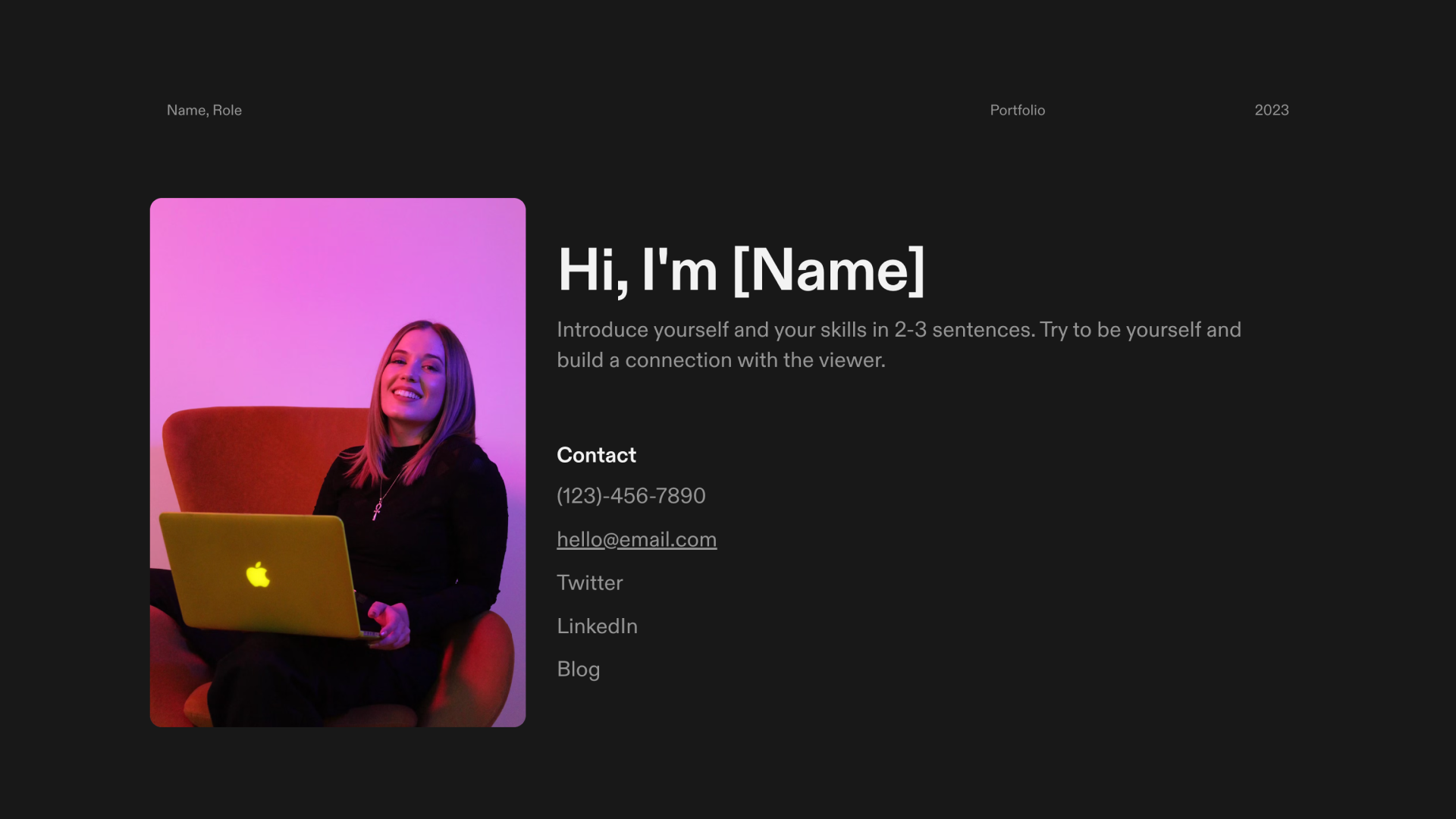
Product Design Portfolio
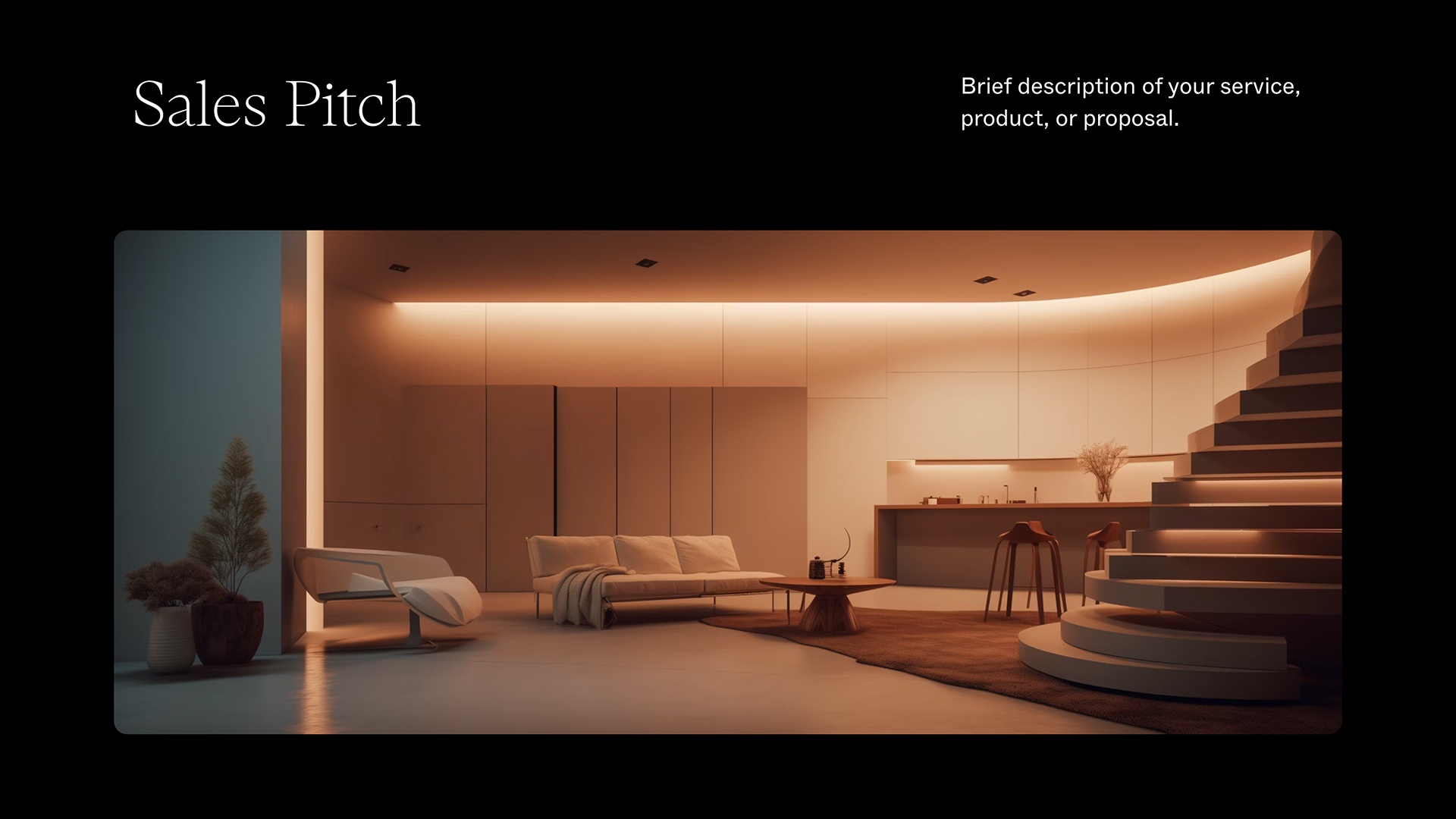
Sales Pitch
Craft your next great idea..
30+ Best Research Presentation Templates for PowerPoint (PPT)
Finding the right PowerPoint template plays an important part in getting your message across to the audience during a presentation. And it’s especially true for research presentations.
Using the right colors, graphs, infographics, and illustrations in your slides is the key to delivering information more effectively and making your presentation a success.
Today, we handpicked a great collection of research presentation PowerPoint templates for you to make the perfect slideshows for various types of research papers and studies.
Whether you’re preparing for a presentation at a school, event, or conference, there are templates in this list for all purposes. Let’s dive in.
2 Million+ PowerPoint Templates, Themes, Graphics + More
Download thousands of PowerPoint templates, and many other design elements, with a monthly Envato Elements membership. It starts at $16 per month, and gives you unlimited access to a growing library of over 2,000,000 presentation templates, fonts, photos, graphics, and more.
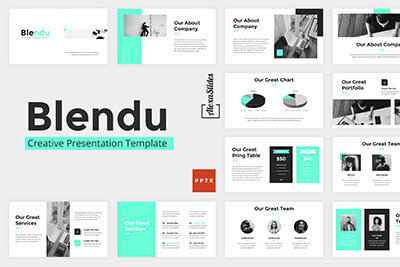
Ciri Template
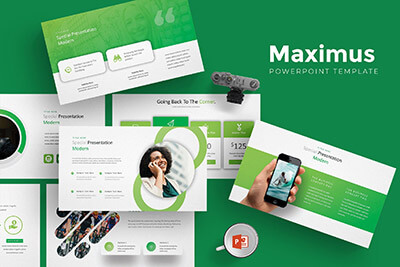
Maximus Template
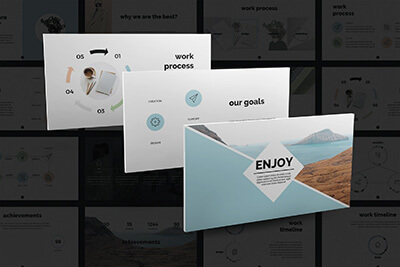
Animated PPT Templates
Fully animated.
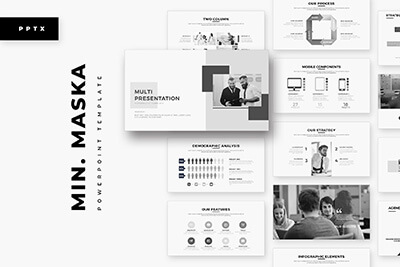
Minimal PPT Templates
Clean & clear.
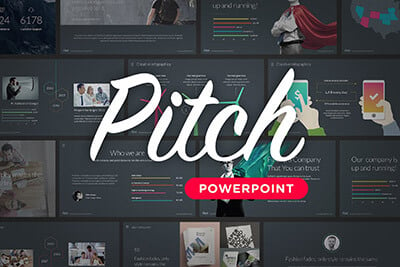
Pitch PowerPoint
Explore PowerPoint Templates
Science & Research Presentation PowerPoint Template
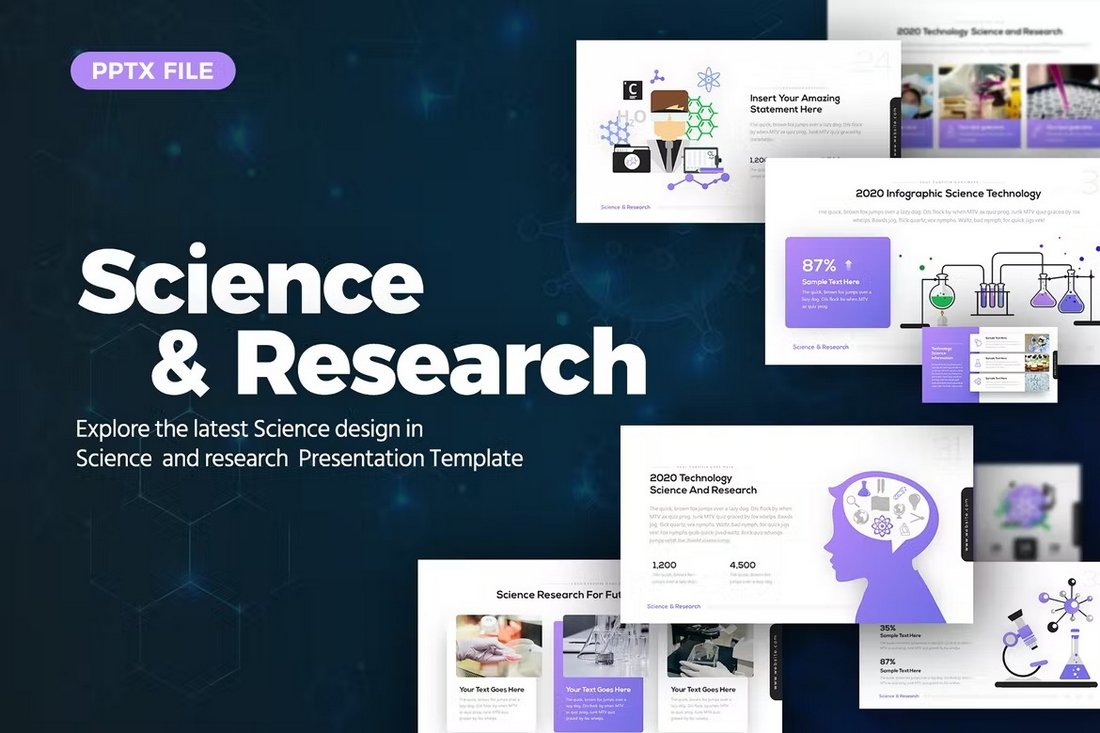
This PowerPoint template is a perfect choice for preparing a research presentation to share your scientific findings and reports.
The template has 30 unique slides with unlimited color options. There are a few infographics included in the slideshow as well.
Why This Is A Top Pick
The presentation has a very modern and creative design where you can showcase your data and information in an attractive way. You won’t be making boring research presentations ever again.
Labvire – Research Presentation PowerPoint Template
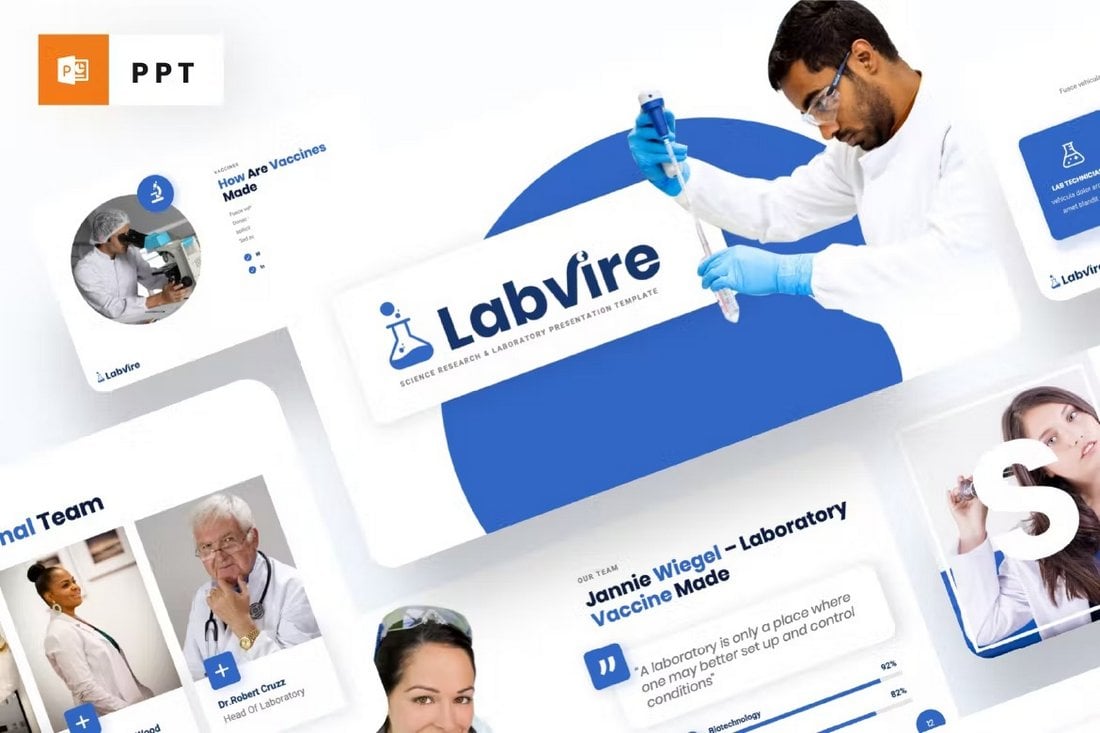
Labvire is another modern PowerPoint template you can use for various types of research presentations. It’s also ideal for laboratory-related research presentations. The template has fully customizable slide layouts with editable charts, graphs, and more. You can choose from more than 40 unique slide designs as well.
Novalabs – Science Research PowerPoint Template
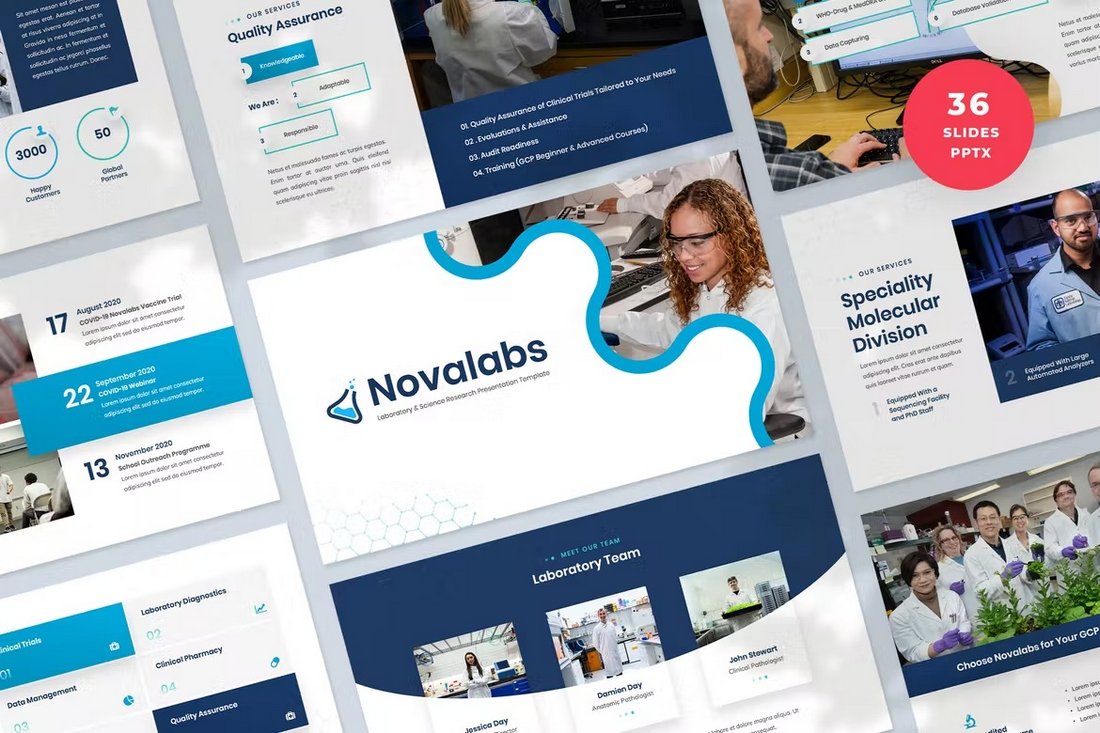
Novalabs PowerPoint template features a highly visual and attractive design. The template includes 36 different slides that feature large image placeholders for adding a more visual look to your presentations. There are lots of editable graphics, shapes, and tables included in the template too. Feel free to customize them however you like.
Research & Development PowerPoint Template
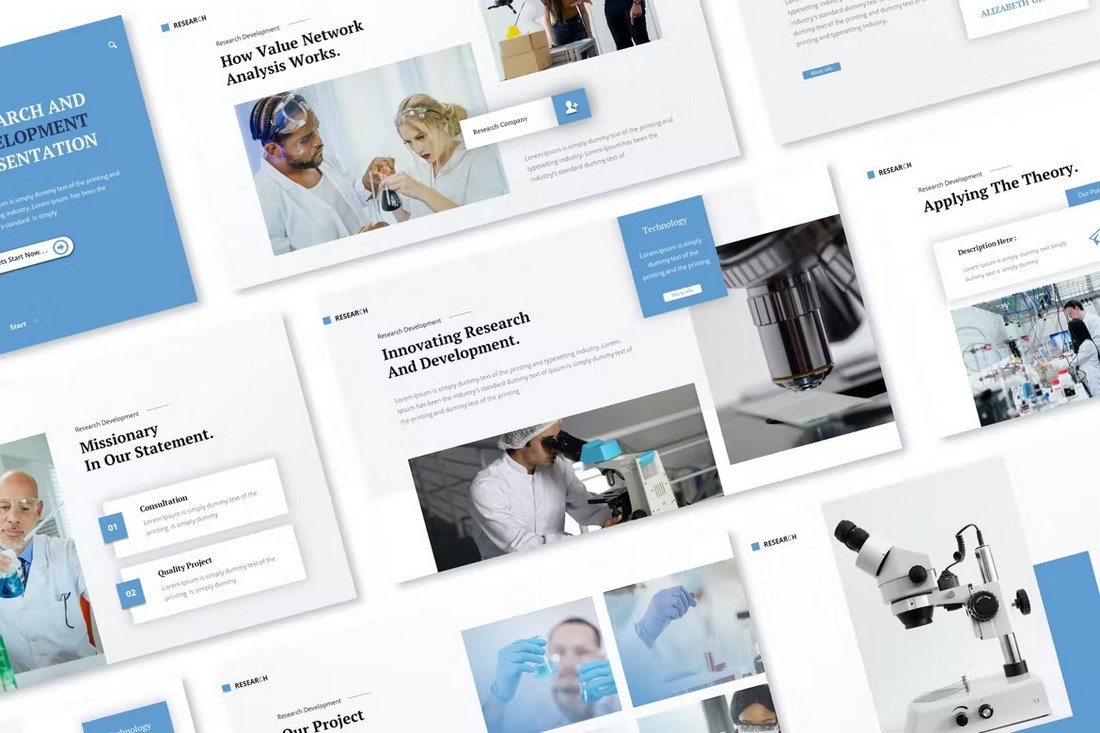
The minimal and clean design of this PowerPoint template makes it a great choice for delivering more effective research presentations. With fewer distractions in each slide, you’ll be able to convey your message more easily. The template comes with 30 unique slides. You can change the colors, fonts, and shapes to your preference as well.
Marketing Research Presentation PowerPoint Template
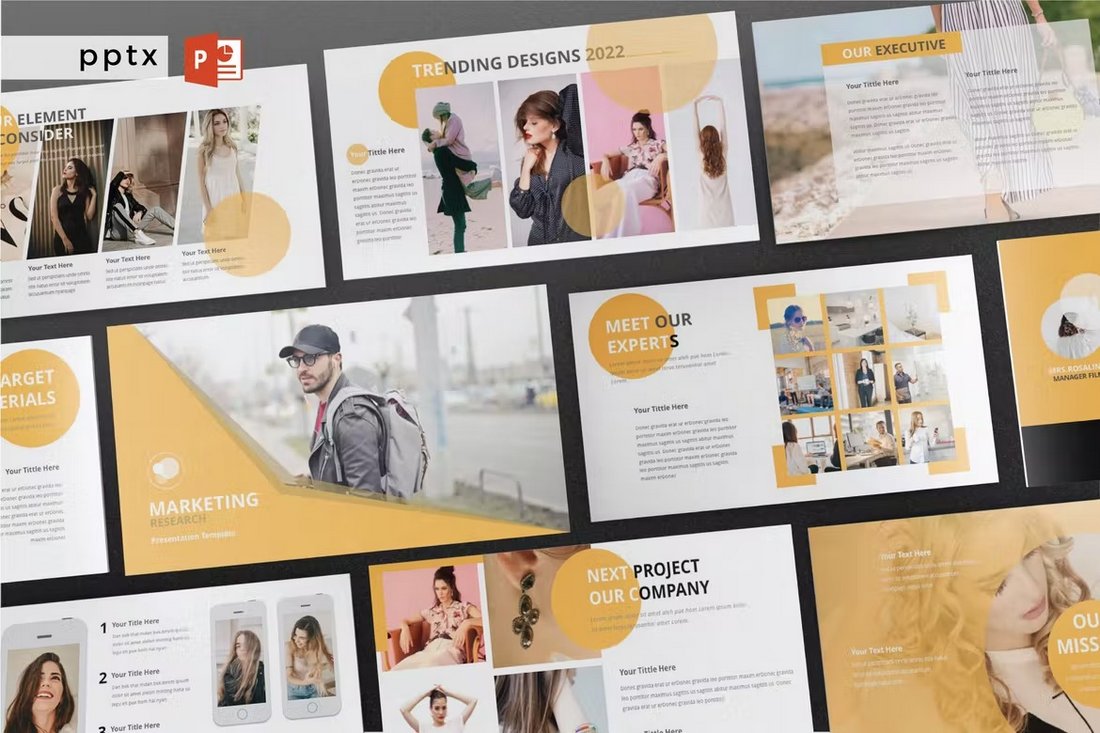
When talking about research presentations, we can’t forget about marketing research. Most sales and marketing meetings usually include a sophisticated marketing research presentation. This PowerPoint template will help you design those research presentations without effort. It includes a total of 150 slides, featuring 30 unique slides in 5 different color schemes.
Free Business Market Research Presentation Template
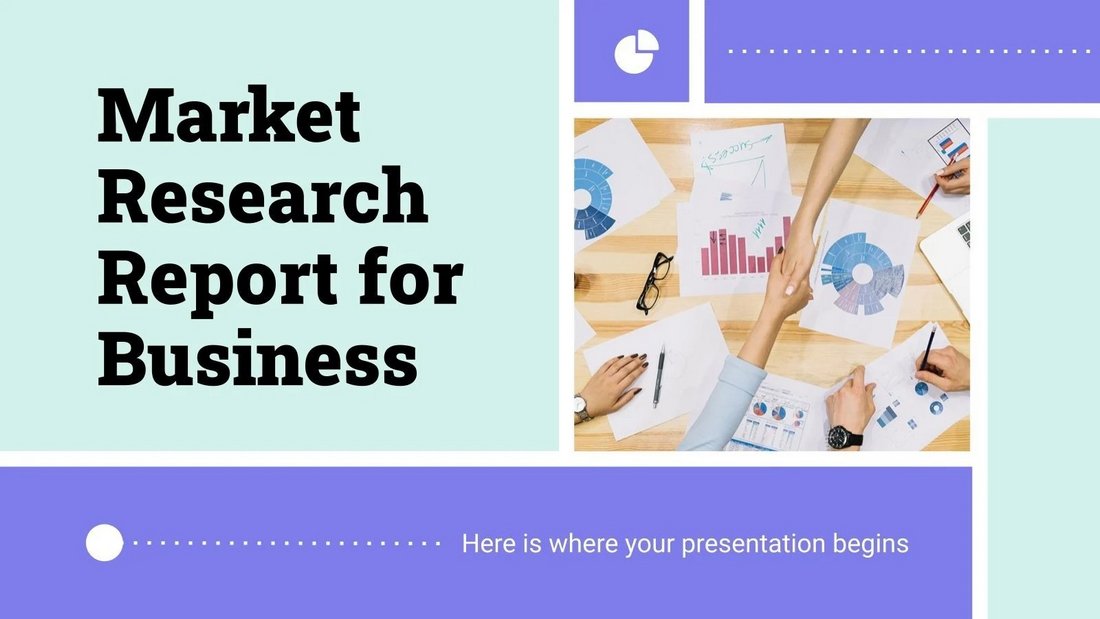
This is a free PowerPoint template designed for making business market research presentations. It gives you 27 different and fully customizable slides to create professional slideshows for your business meetings.
Free Business Data Analysis & Research Presentation
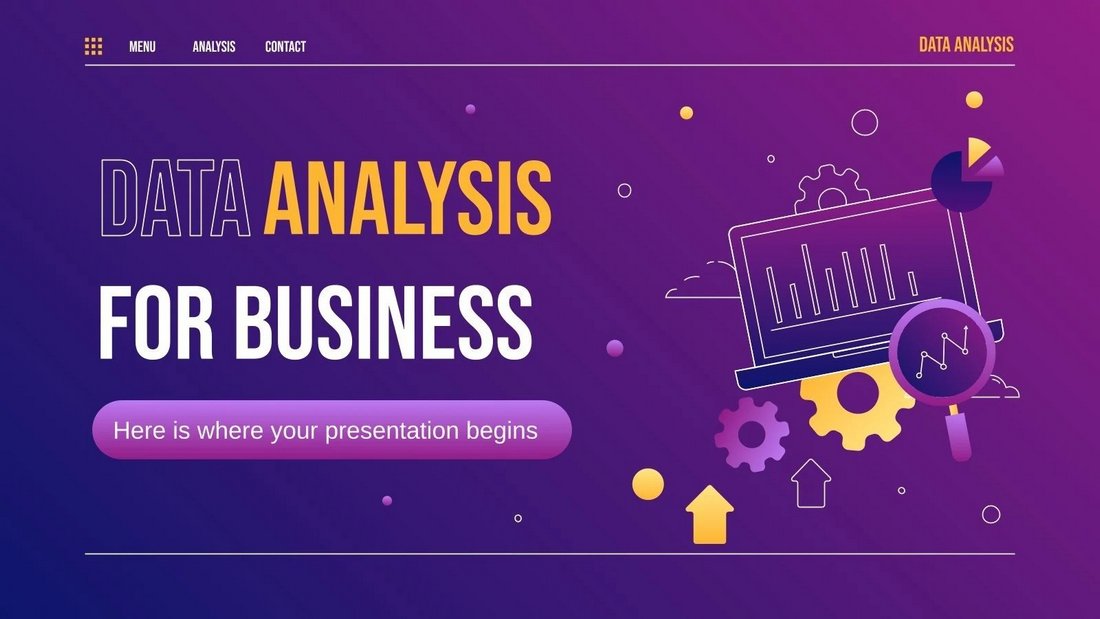
With this PowerPoint template, you can create colorful and creative business research and data analysis presentation without any design skills. It includes 35 unique slides with lots of infographics and editable shapes. The template is free to use as well.
Lernen – Research Thesis PowerPoint Presentation
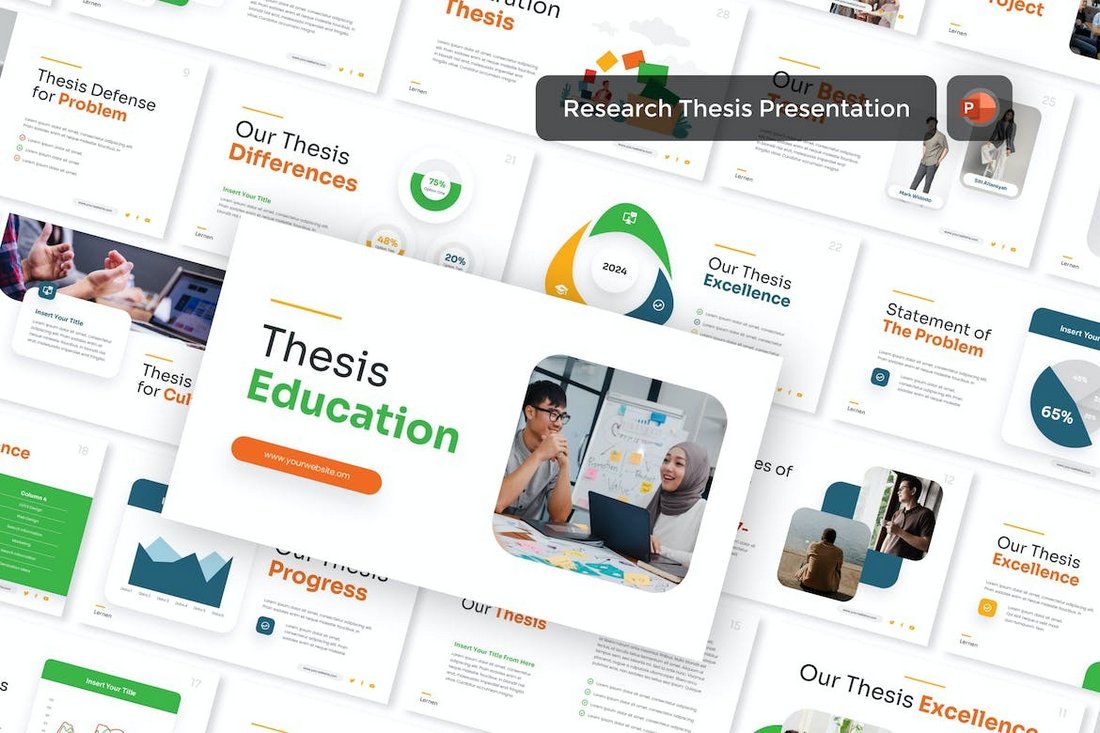
Larnen is the ideal PowerPoint template for making research slideshows for your thesis presentations. It includes 30 unique slides that are available in light and dark color themes. It also has editable charts and graphs.
Aristo – Research Academic PowerPoint Presentation
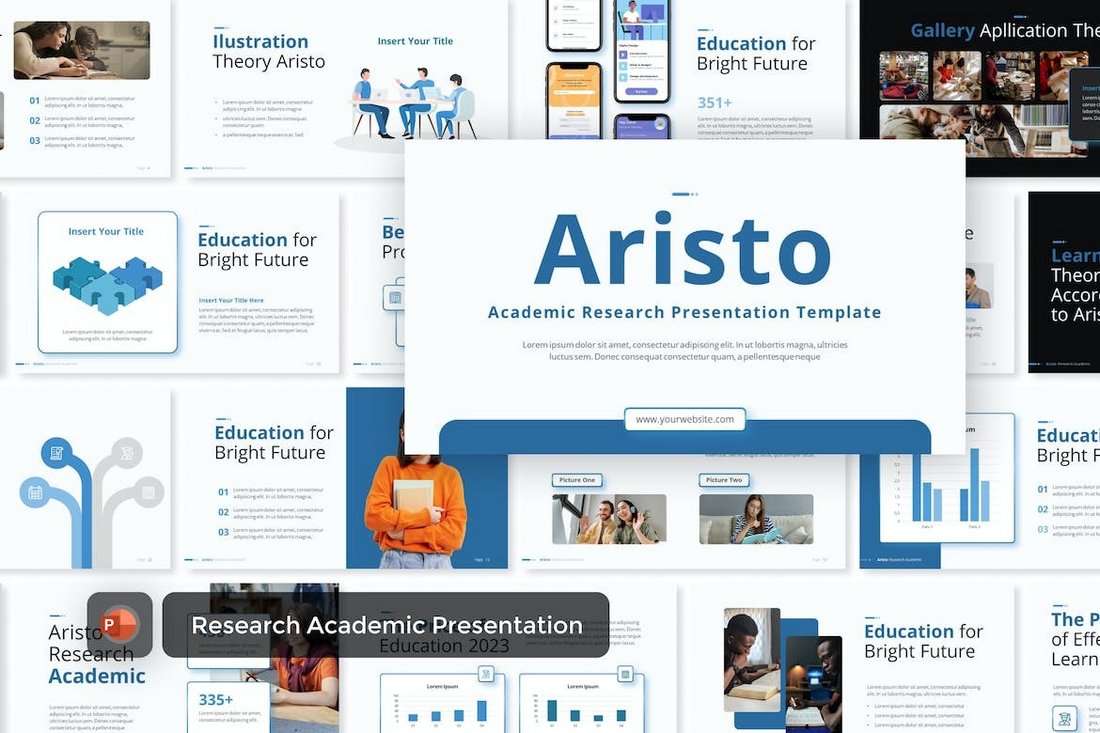
This PowerPoint template is also made with academic research presentations in mind. The template has a professional design with clean layouts and light colors. It comes with more than 30 different slides.
Biosearch – Science Research PowerPoint Template
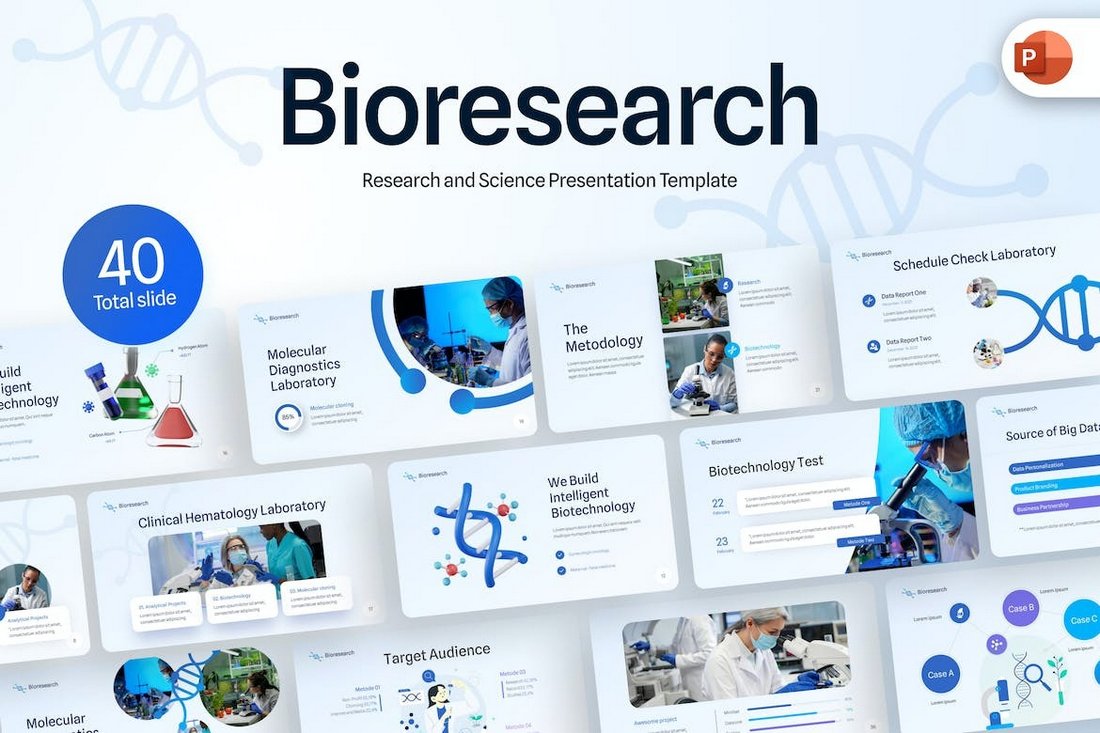
You can use this PowerPoint template to make professional presentations to present research data and results. It lets you choose from 40 different slides and 90 color themes. The slides are available in both light and dark color themes as well.
Neolabs – Laboratory & Science Research PPT
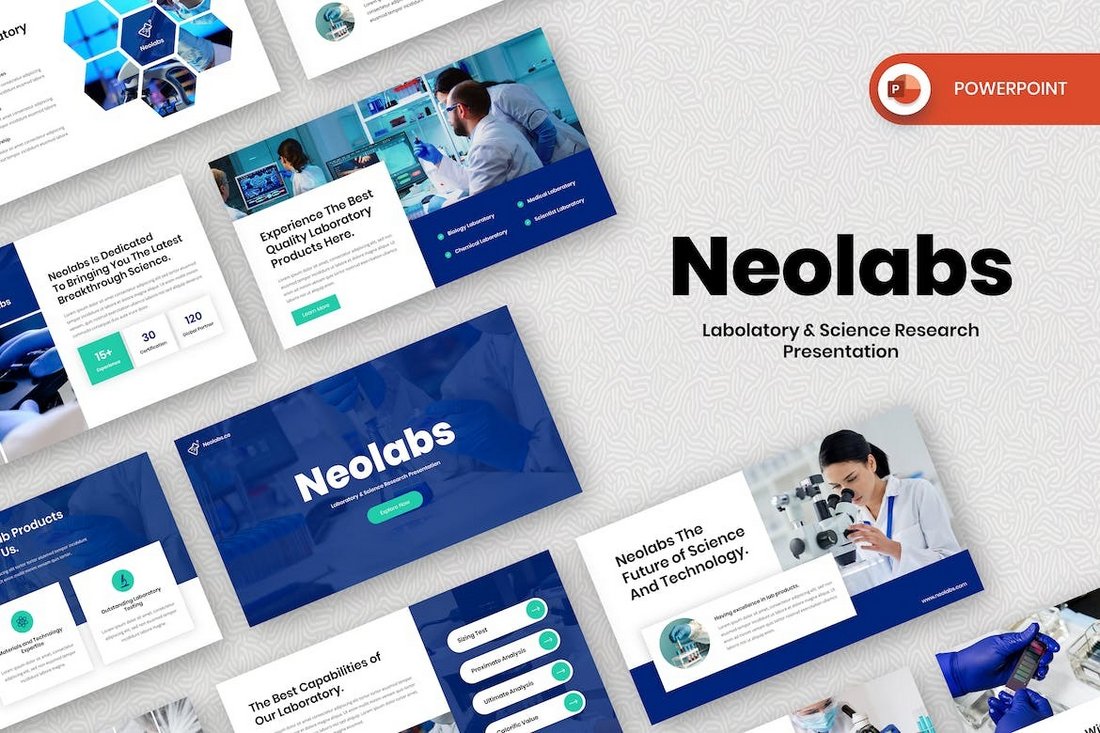
Neolabs is another science research presentation made with laboratory research teams in mind. You can use it to make effective slideshows to present your research findings. There are 30 unique slides in this template.
Free Business Cost Analysis PowerPoint Template
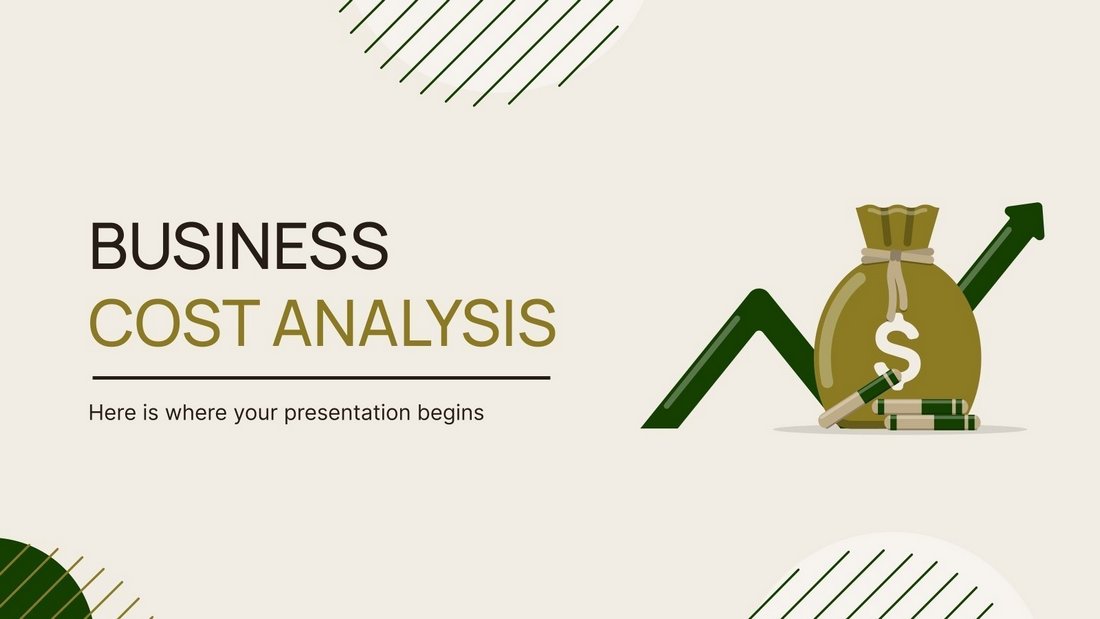
This is a free PowerPoint and Google Slides template that comes with 35 unique slides. It’s ideal for making research presentations related to business financials.
Research & Case Study PowerPoint Template
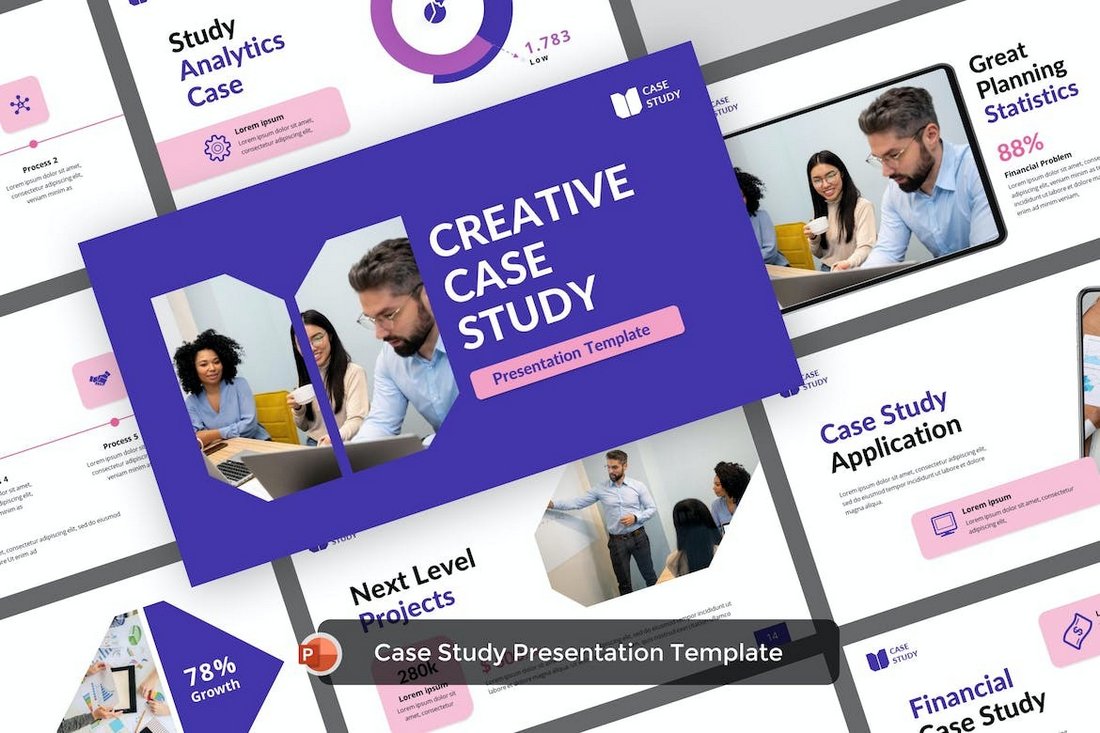
Create the perfect case study presentation using your research data with this PowerPoint template. It includes a modern slide design with infographics and charts for effectively presenting your data.
Liron Labs – Laboratory Research PowerPoint Template
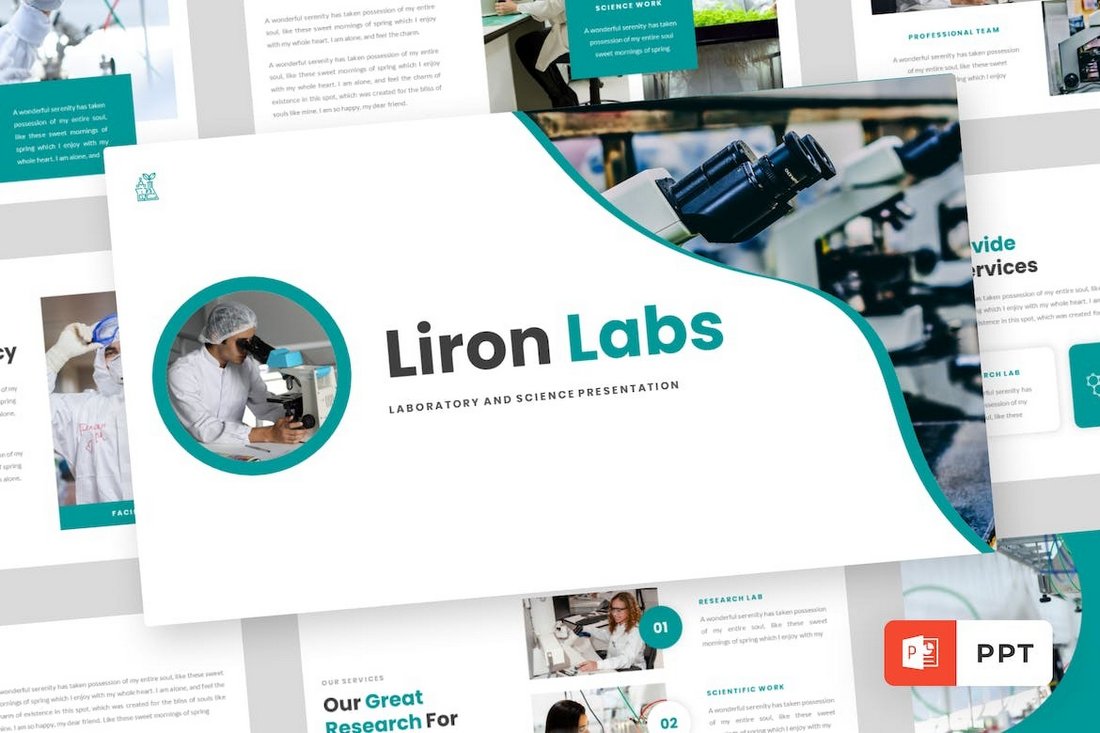
Another PowerPoint template for laboratory research presentations. This template includes 15 useful slide layouts with editable graphics, free fonts, and image placeholders. You can edit and customize the colors and text as well.
Research Thesis PowerPoint Template
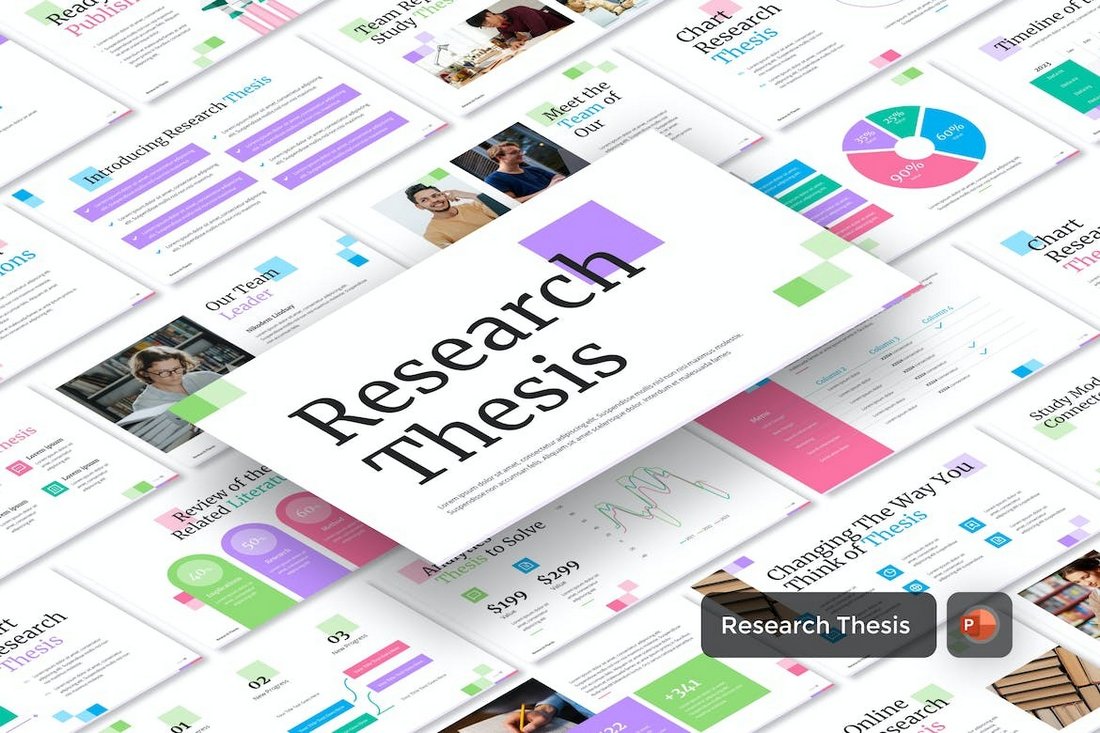
Make an attractive and creative research thesis presentation using this PowerPoint template. There are over 30 unique slides in this template. You can either use dark or light color themes to create your presentations.
Colorful Thesis Research PowerPoint Template
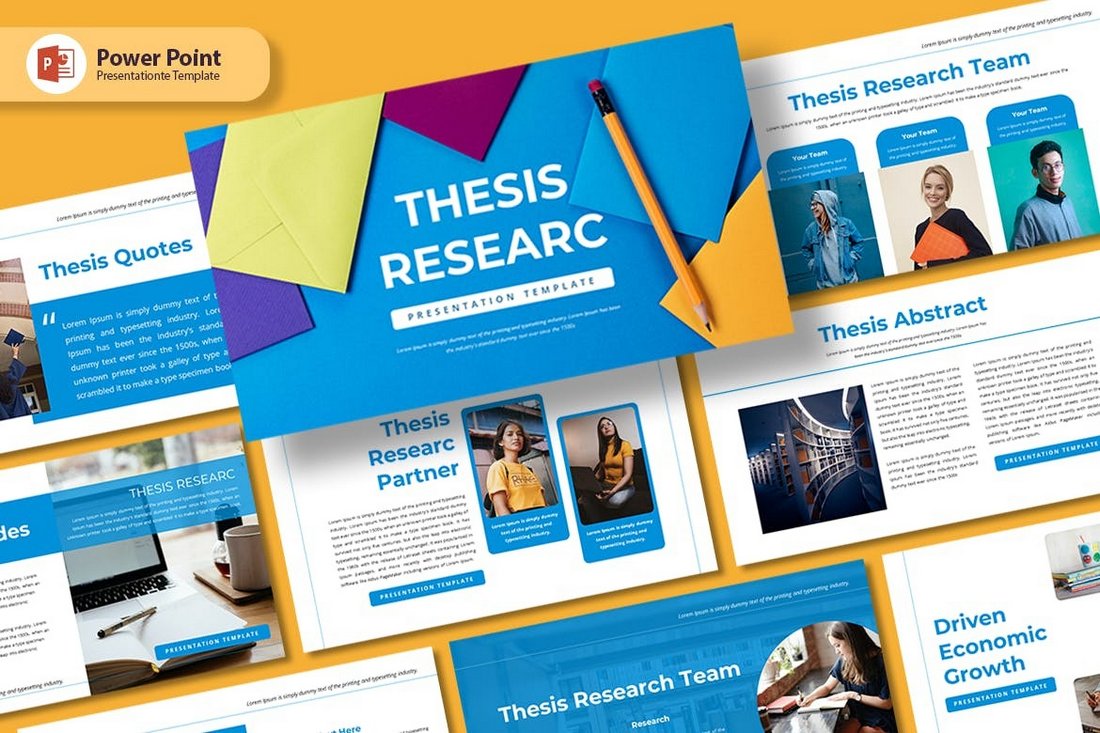
If you want to make your research presentations look more colorful and creative, this PowerPoint template is for you. It has 15 different slides with fully customizable layouts. It has editable shapes, free fonts, and image placeholders too.
Free Data Analysis Research PowerPoint Template
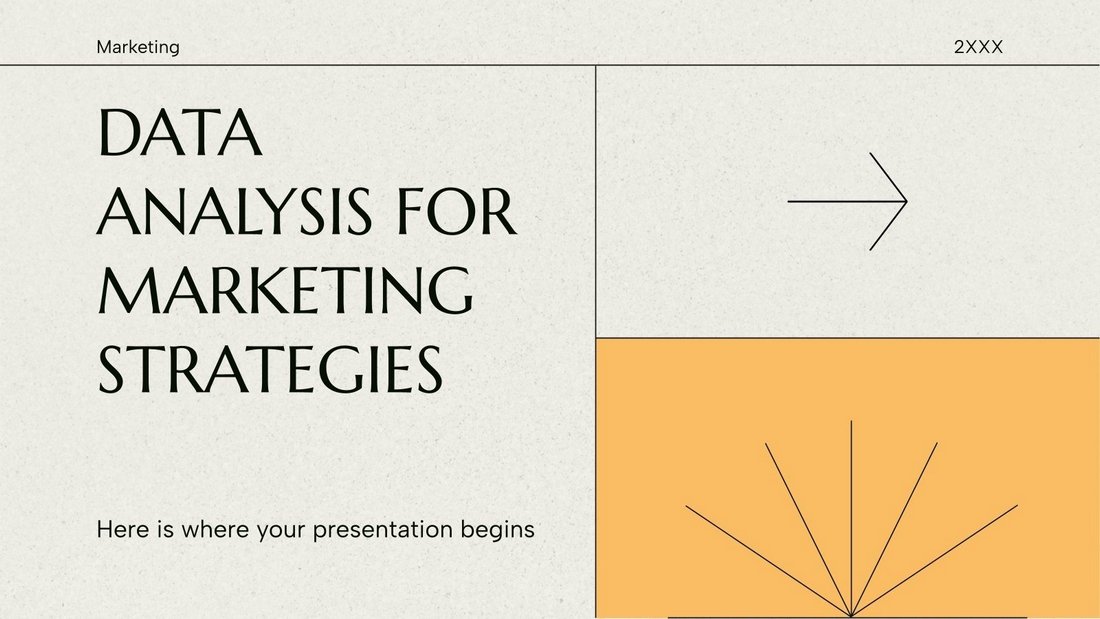
This PowerPoint template is also free to download. You can also customize it using PowerPoint or Google Slides. This template is ideal for marketing agencies and teams for presenting research and data analysis.
Laboratory & Science Research PowerPoint Template
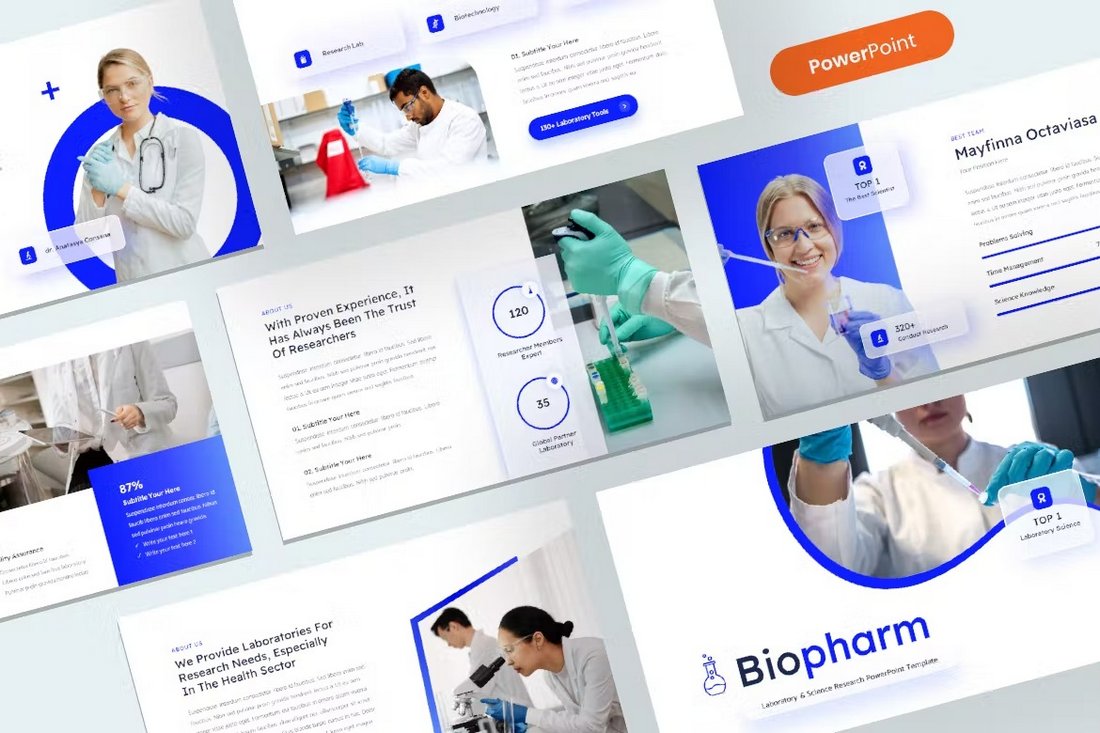
You can make more convincing and unique lab research presentations using this PowerPoint template. It features a creative design that will easily attract the attention of your audience. You can use it to make various other science and research presentations too. The template includes 30 unique slides.
The Biologist – Research Presentation PowerPoint Template
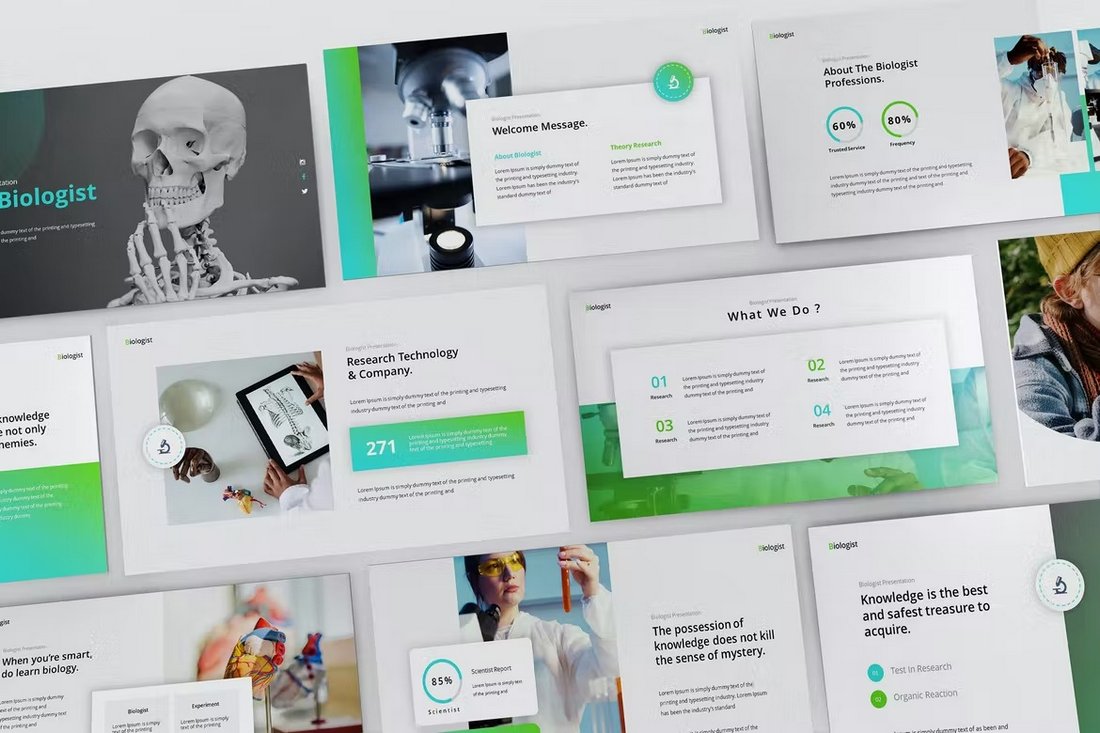
Just as the name suggests, this PowerPoint template is designed with biology and science-related presentations in mind. It includes many useful slide layouts that can be used to make various types of research presentations. There are 30 different slide designs included in this template with editable shapes and colors.
Modern Science & Research PowerPoint Template
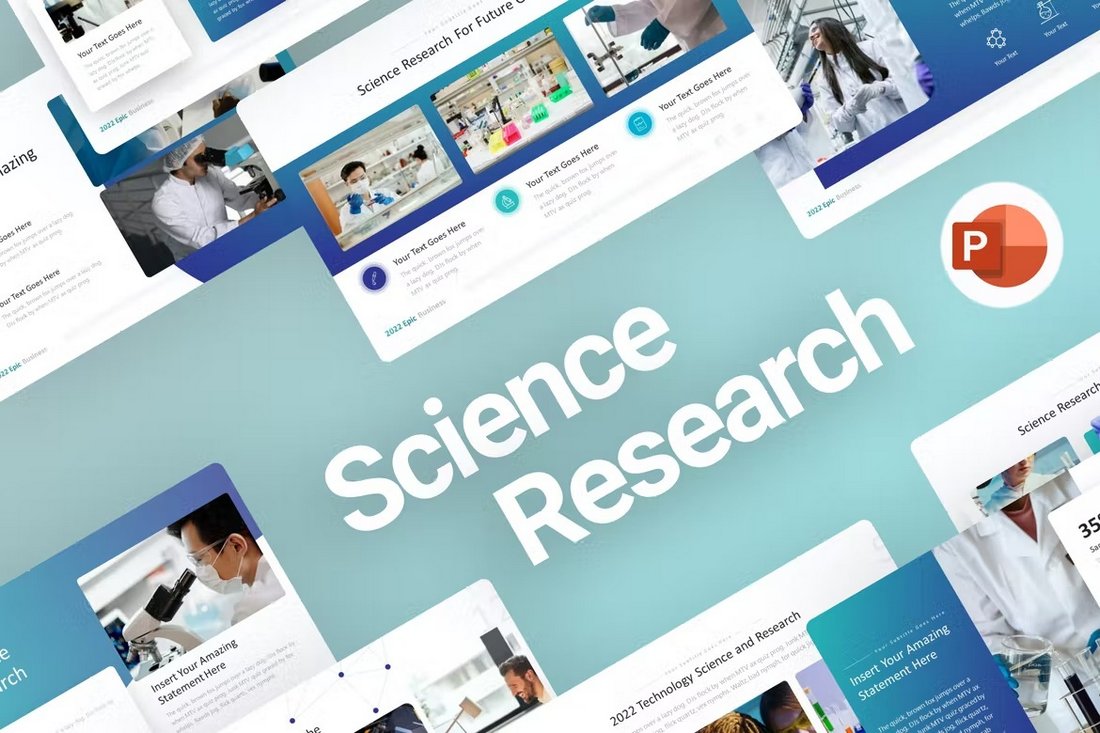
If you’re looking for a PowerPoint template to create a modern-looking research presentation, this template is perfect for you. It features a collection of modern and attractive slides with lots of space for including images, icons, and graphs. There are 30 unique slides in the template with light and dark color themes to choose from.
Marketing Report & Research PowerPoint Template
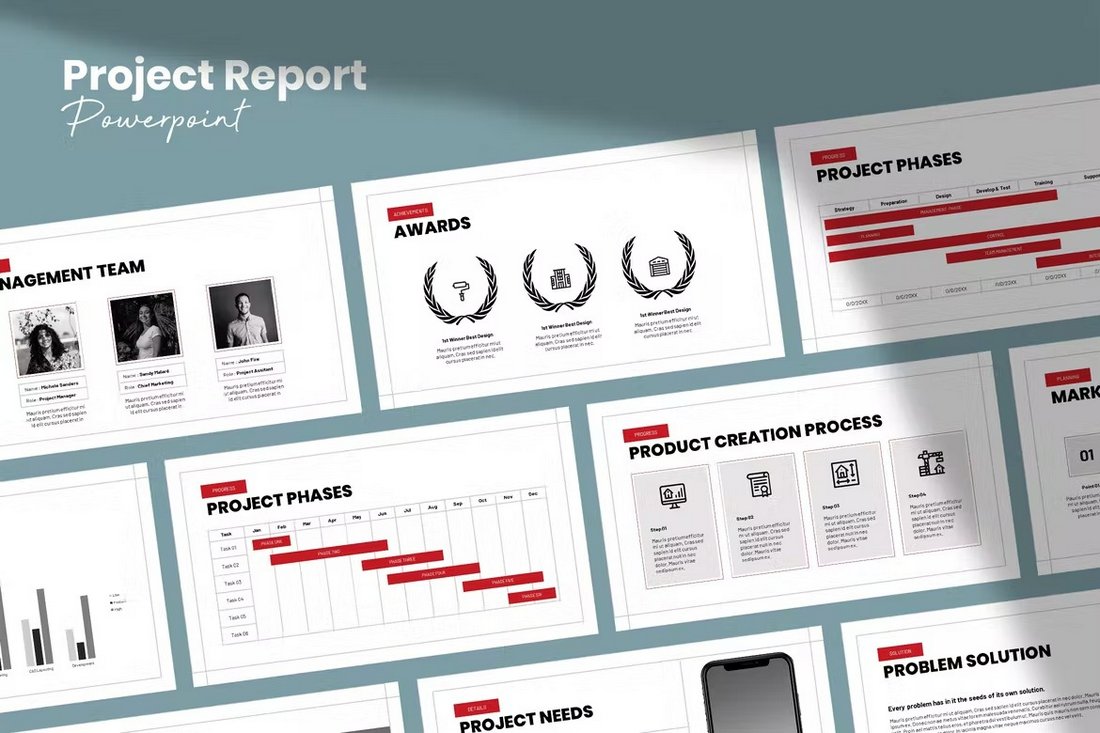
This PowerPoint template doubles as both a research and report slideshow. You can use it to create various marketing reports as well as marketing research presentations. It comes with 30 slides that feature minimal and clean designs. It includes lots of editable charts, infographics, and tables as well.
Market Research Presentation PowerPoint Template
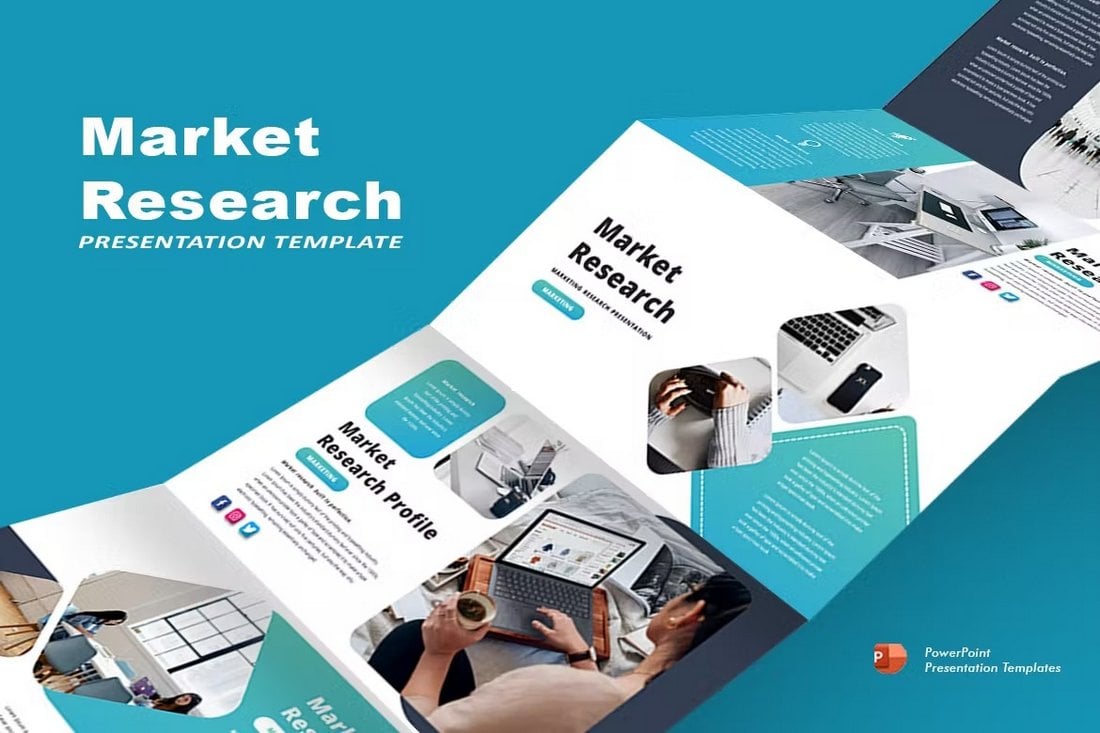
Another modern PowerPoint template for making market research presentations. This template includes 25 unique slides with master slides, image placeholders, and editable colors. The template is ideal for marketing agencies and corporate businesses.
Free Academic Research Thesis PowerPoint Template
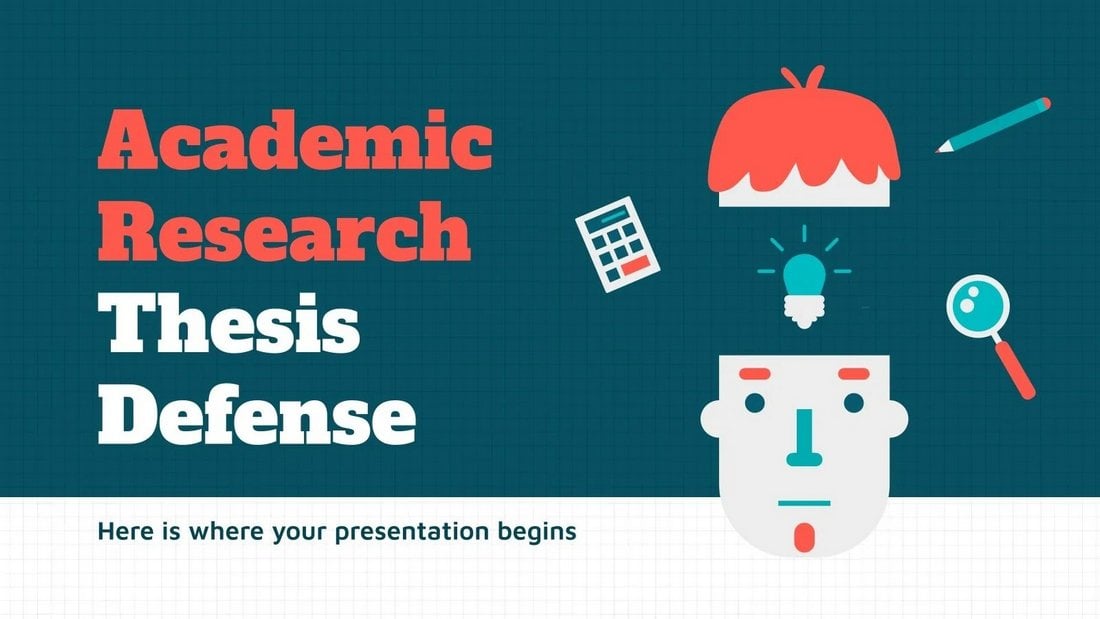
This free PowerPoint template is designed for defending your academic research thesis dissertation. Needless to say, it’s a useful template for academics as well as teachers. The template features 23 unique slide layouts with customizable designs.
Free Economics Research Thesis Presentation Template
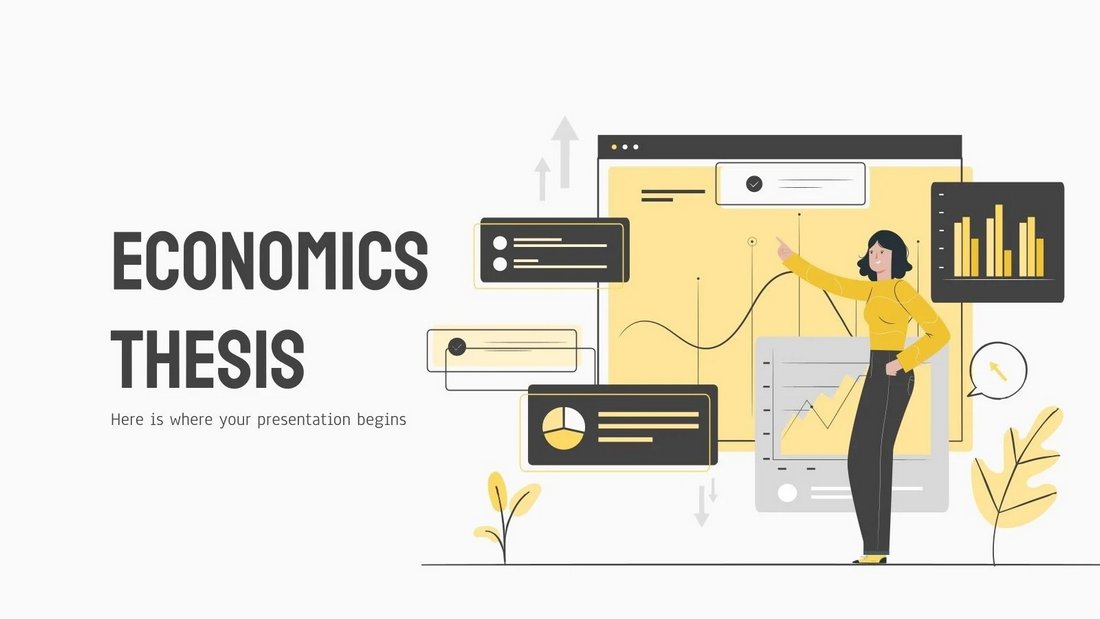
You can use this free template to create thesis and research presentations related to economics. It’s useful for academic students and gives you the freedom to choose from 21 slide layouts to make your own presentations.
Labia – Research Presentation Powerpoint Template
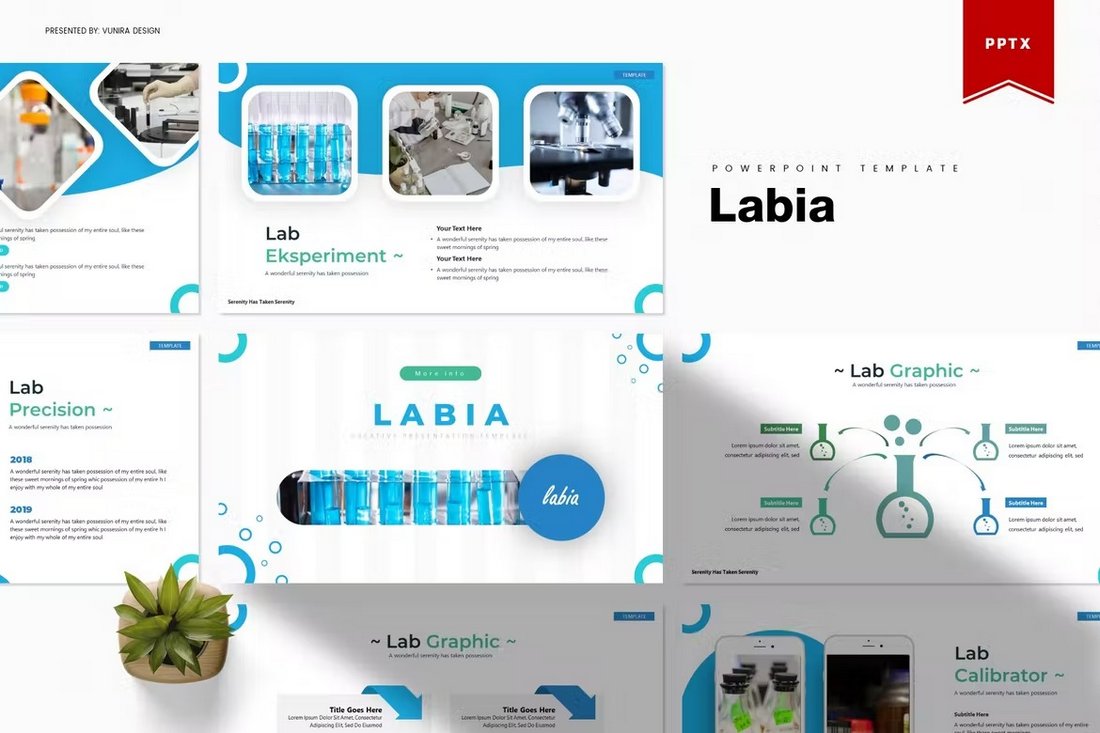
Labia is a research presentation template made for professionals. It comes with a set of modern slides with multipurpose designs. That means you can customize them to make many different types of research presentations. There are 30 unique slides included in this template that come in 5 different color themes.
Medical Research Infographics & Powerpoint Slides
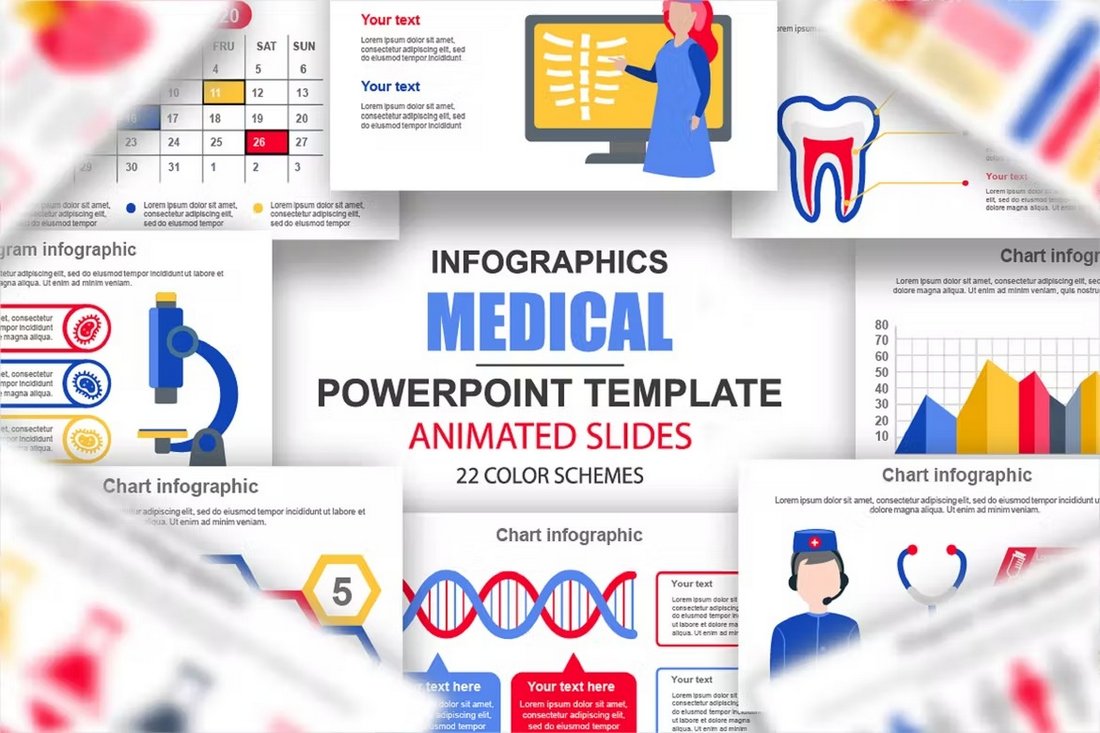
You’ll be using lots of charts, graphs, and infographics in your presentations to showcase data in visual form. Not to mention that visuals always work well for attracting the audience’s attention. You can use the infographic slides in this template to create better research presentations. Each slide features a unique infographic with animated designs.
Foreka – Biology Education & Research Presentation PPT
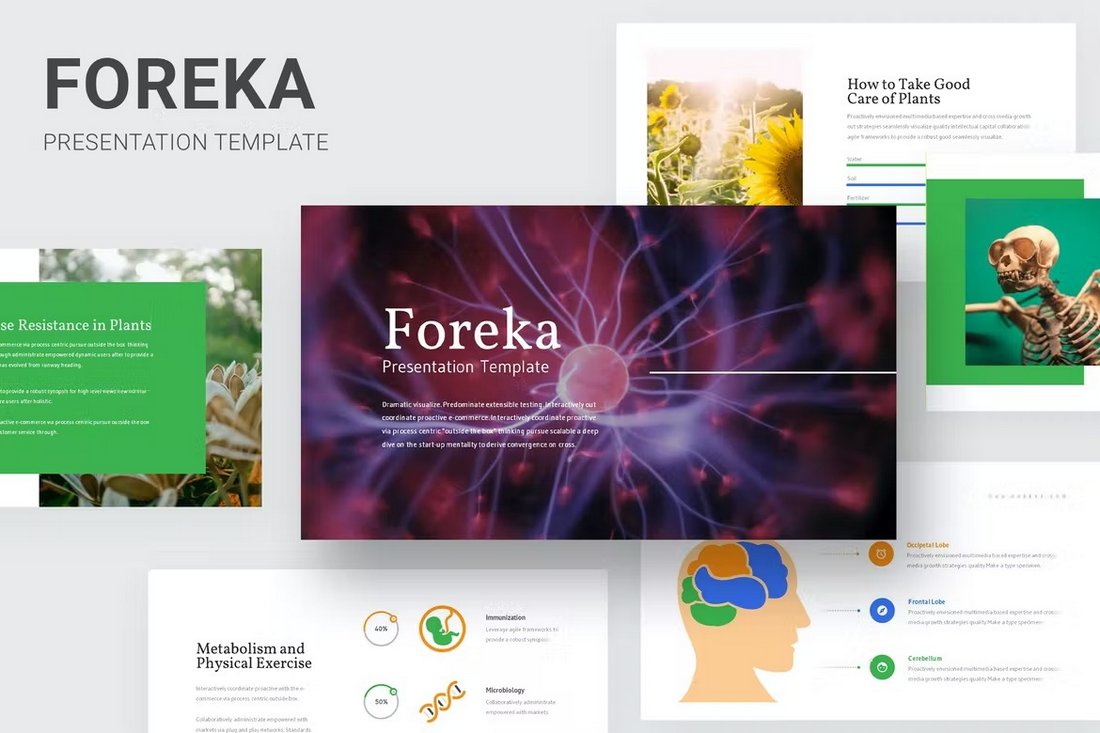
Foreka is a PowerPoint template made for educational presentations, especially for covering topics related to biology. But it can also be customized to present your research presentations. The slides have very useful layouts that are most suitable for making research slide designs. There are 30 slides included with light and dark color themes.
Maua – Aesthetic Business Research PowerPoint Template
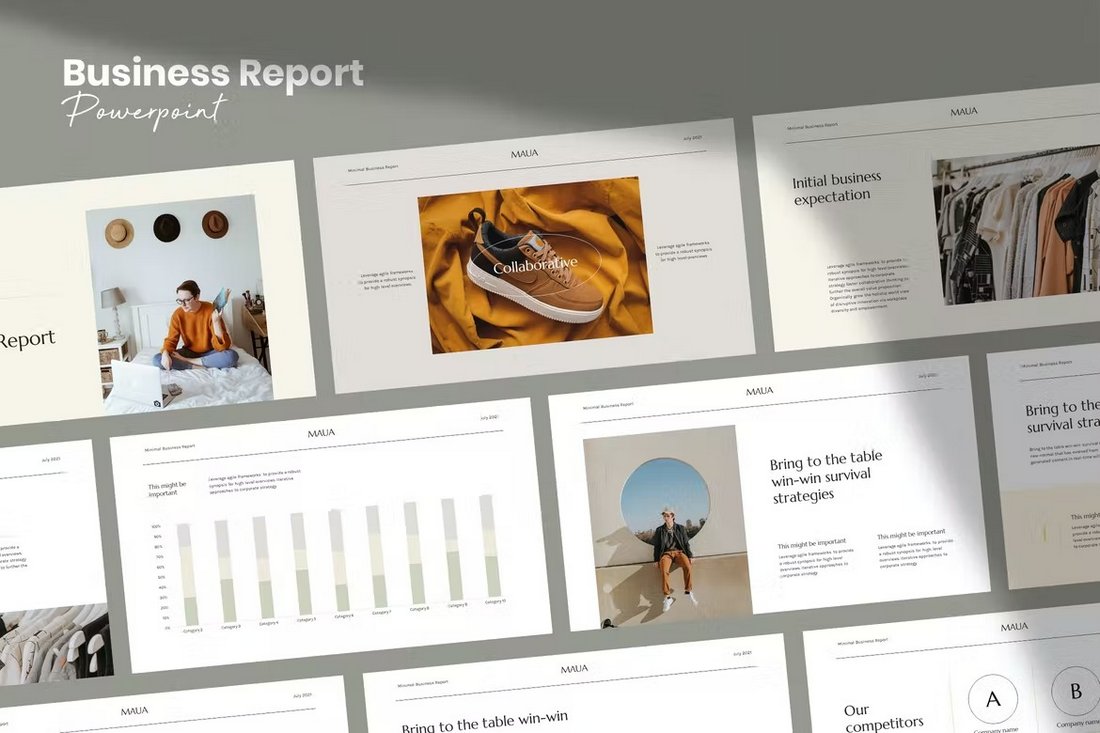
This PowerPoint template is suitable for making elegant and stylish business reports and business research presentations. It’s especially great for making background research and competitor research slideshows. The template comes with 30 slides featuring master slides, image placeholders, and more.
World Data Scientist Powerpoint Presentation Template
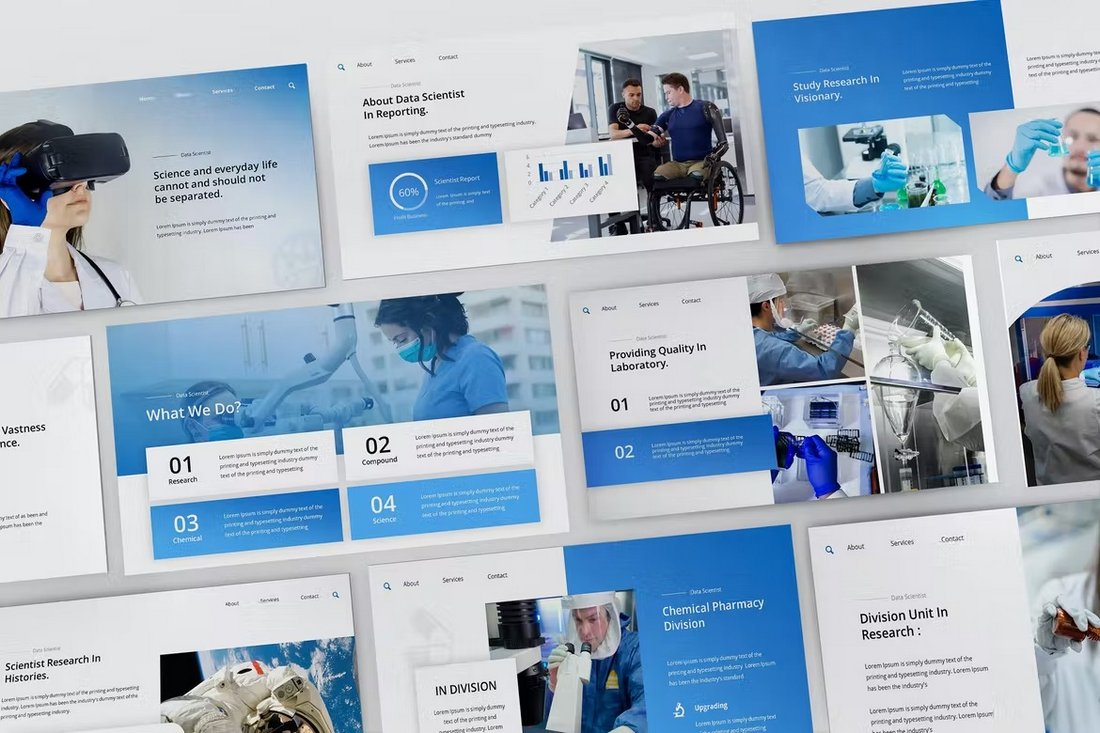
You can use this PowerPoint template to create research presentations for many different types of topics, industries, and projects. The template includes lots of data-centric slides where you can easily showcase your data in visual form. There are 30 unique slides included with the template as well.
Free SWOT Analysis Infographics PowerPoint Template
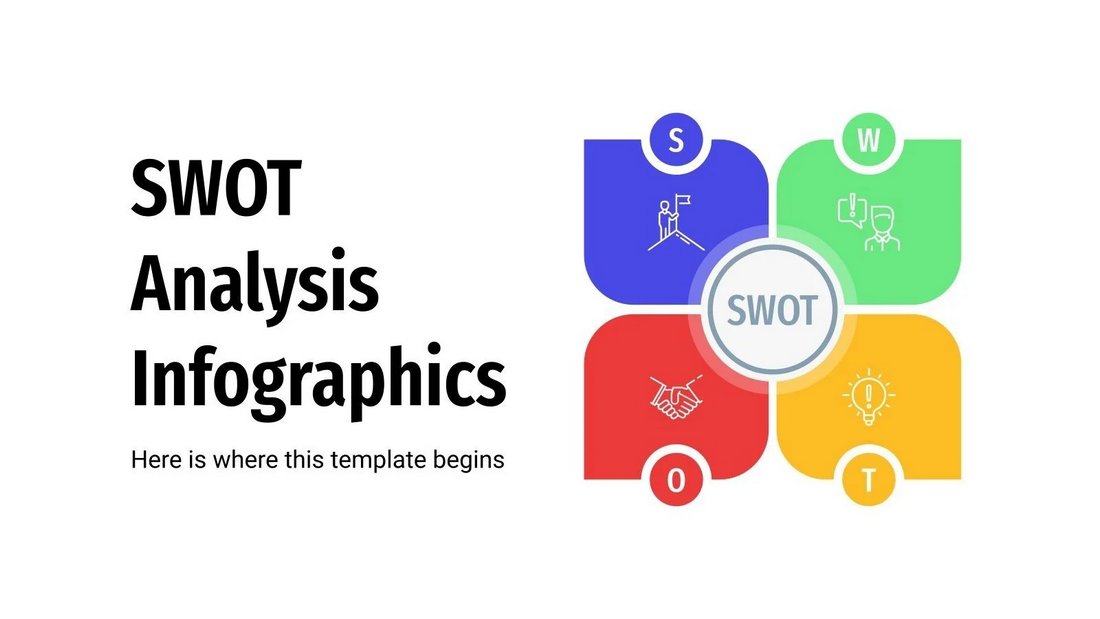
SWOT analysis is a commonly used methodology in business research presentations. With this free PowerPoint template, you can create stylish SWOT analysis infographics for your presentations. It includes SWOT infographics in 30 different styles.
Free Market Research Presentation Infographics PPT
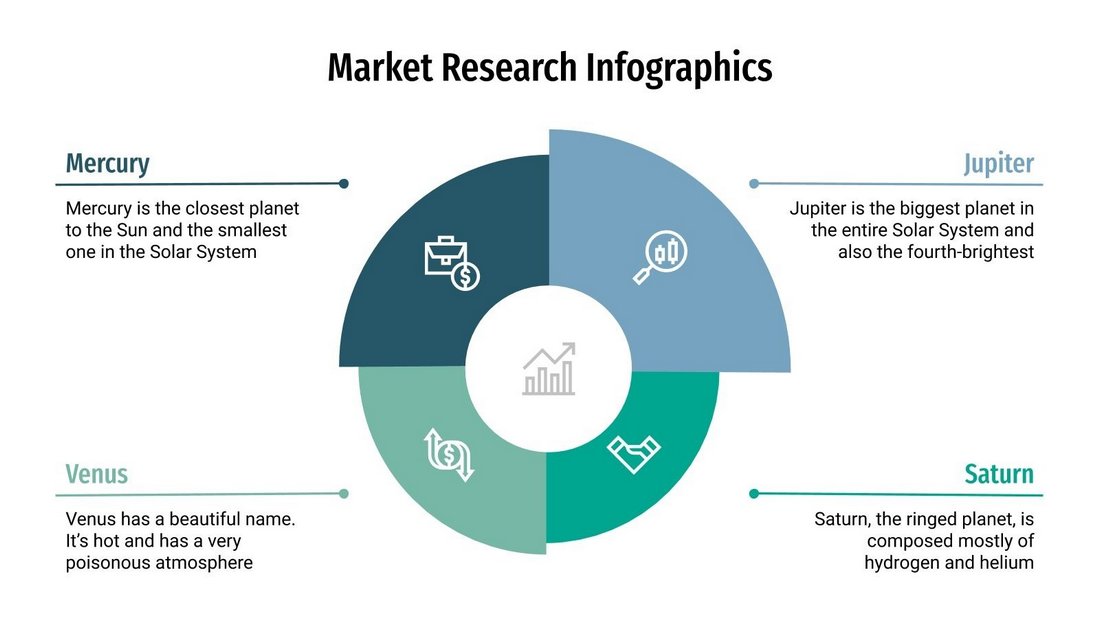
This is a collection of free PowerPoint slides that feature various styles of infographics you can use in your business and market research presentations. There are 30 different infographic slides included in this template. You can edit, change colors, and customize them however you like.
Sinara – Science & Research Powerpoint Template
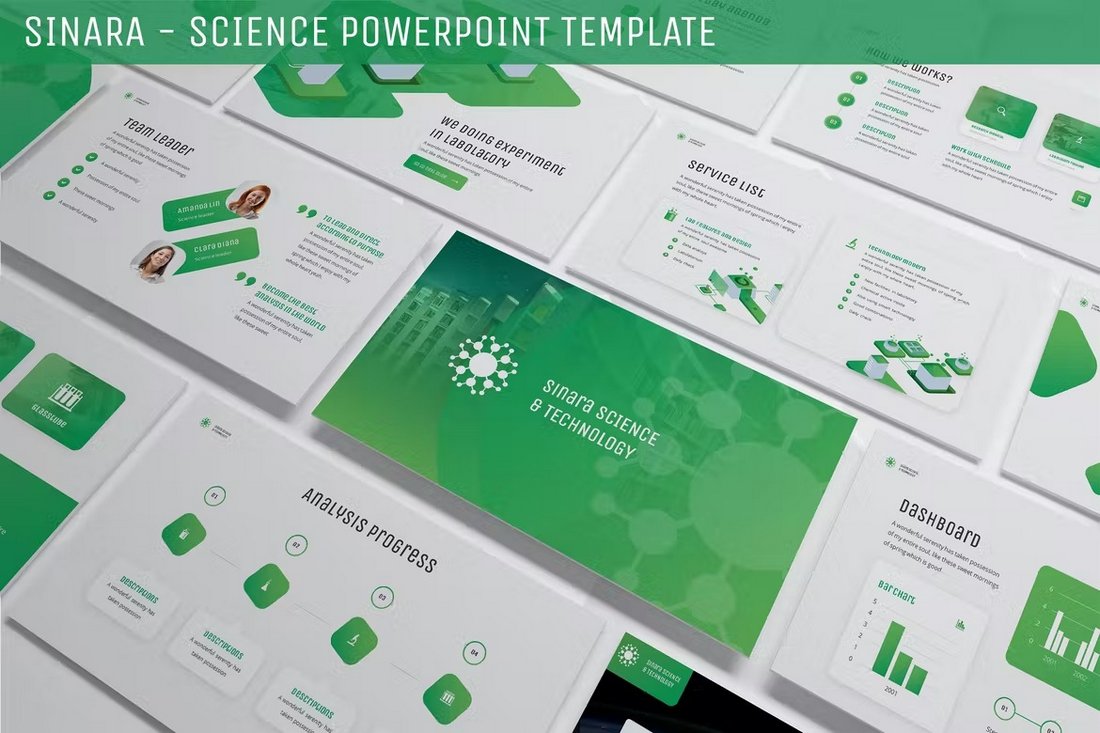
Sinara is a brilliant PowerPoint template you can use to craft a professional presentation for science-related research and reports. It’s available in 3 different color schemes as well as the option to customize the colors to your preference. The template comes in light and dark themes too.
Political Science and Research PowerPoint Template
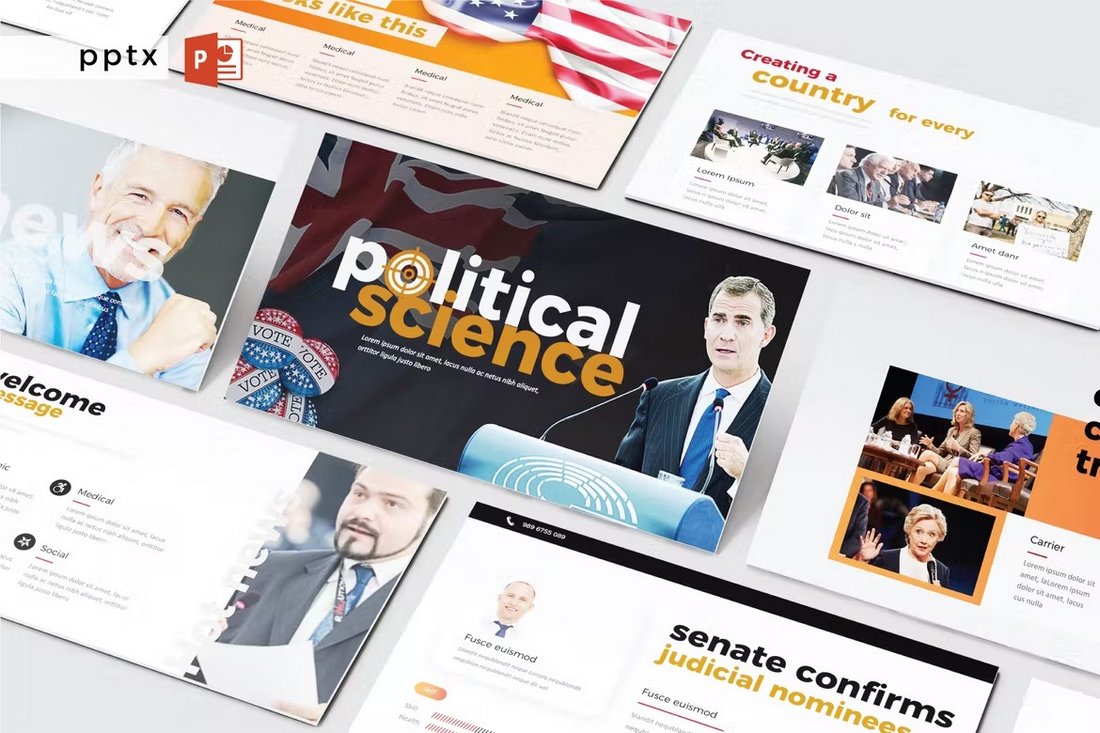
This PowerPoint template will be quite useful to political science and international relations students. It features a total of 150 slides you can use to create attractive presentations for your research and methodologies. There are slides in 5 different color schemes.
How to Make a Research Poster in PowerPoint
We bet you didn’t know that you could actually design posters in PowerPoint. Well, you can and it’s very easy to do so.
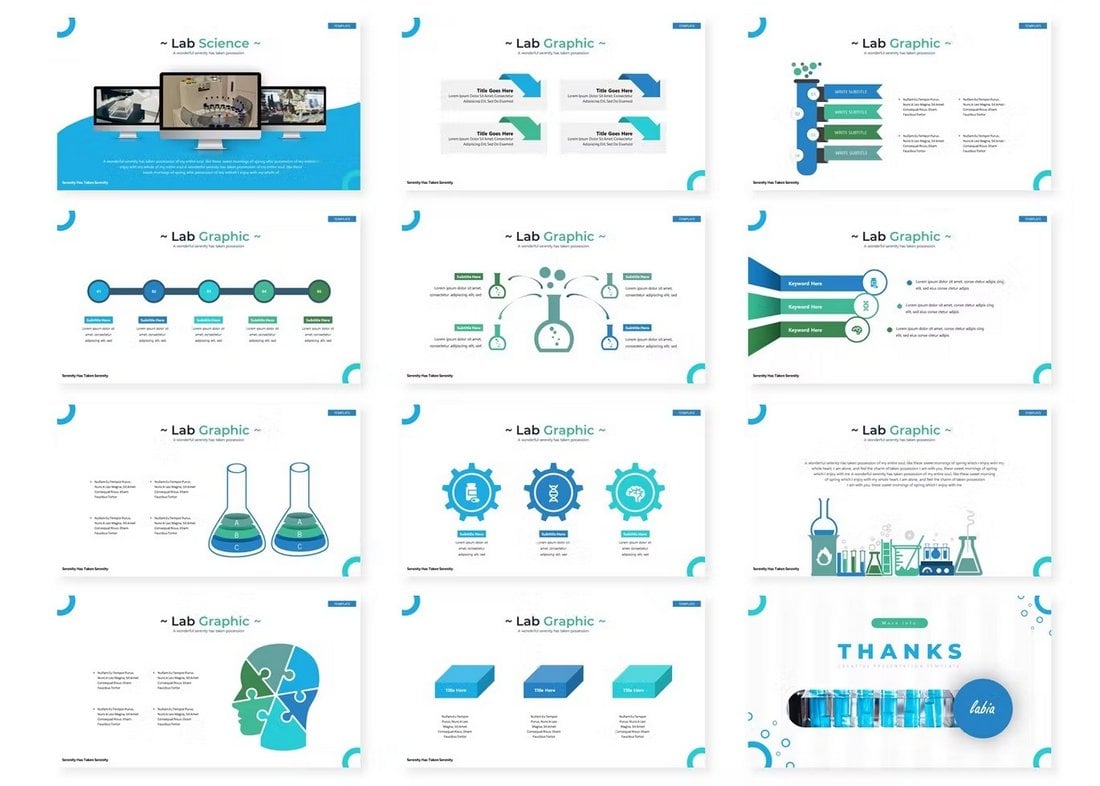
The easiest way to make a poster in PowerPoint is to use a pre-made template like the one above.
You can easily copy one of the slides from a template, and resize the slide dimensions to create a vertical poster. Then add a title with a few lines of text and you’ll have yourself a poster.
Or, if you want to craft a poster from scratch, you can read our complete guide on how to create posters in PowerPoint with step-by-step instructions.
For more useful presentation templates, be sure to check out our best educational PowerPoint templates collection.
tools4dev Practical tools for international development
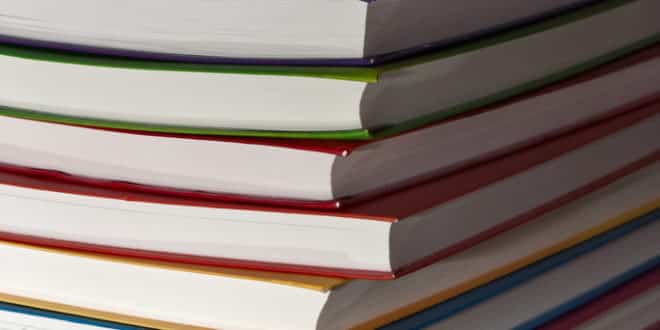
Research report template
A research report describes the results of a survey, interviews, focus groups or any other type of qualitative or quantitative research. Even if they aren’t necessarily “researchers”, most international development practitioners will still need to write a basic research report at some point in their career. Either for a baseline or endline survey, needs assessment, or to describe the results of interviews or focus groups with program participants. This template can be used as a starting point for any type of basic research report (qualitative or quantitative).
Download research report template
This research report template is appropriate when:
- You need to write a report after conducting surveys, interviews, focus groups, or any other type of qualitative or quantitative research.
- You need to write a report for a simple baseline or endline survey, or needs assessment.
This research report template is NOT appropriate when:
- You need to write an academic research report.
- You need to write a report for a very complex or large research project (you could start with this template, but it would need a lot of modifications).
The Research Report Template by tools4dev is licensed under a Creative Commons Attribution-ShareAlike 3.0 Unported License . All other content is © tools4dev .
Photo by Horia Varlan
Tags Monitoring & Evaluation Program Design
About Piroska Bisits Bullen

Related Articles
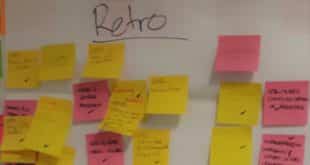
What can international development learn from tech start-ups?
13 May 2021
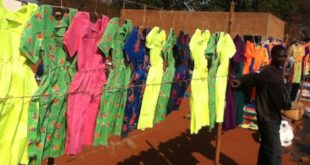
Social Enterprise Business Plan Template
12 May 2021
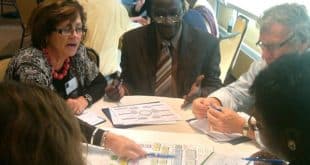
How to write an M&E framework – Free video tutorial & templates
10 September 2017
- Privacy Policy

Home » Research Summary – Structure, Examples and Writing Guide
Research Summary – Structure, Examples and Writing Guide
Table of Contents

Research Summary
Definition:
A research summary is a brief and concise overview of a research project or study that highlights its key findings, main points, and conclusions. It typically includes a description of the research problem, the research methods used, the results obtained, and the implications or significance of the findings. It is often used as a tool to quickly communicate the main findings of a study to other researchers, stakeholders, or decision-makers.
Structure of Research Summary
The Structure of a Research Summary typically include:
- Introduction : This section provides a brief background of the research problem or question, explains the purpose of the study, and outlines the research objectives.
- Methodology : This section explains the research design, methods, and procedures used to conduct the study. It describes the sample size, data collection methods, and data analysis techniques.
- Results : This section presents the main findings of the study, including statistical analysis if applicable. It may include tables, charts, or graphs to visually represent the data.
- Discussion : This section interprets the results and explains their implications. It discusses the significance of the findings, compares them to previous research, and identifies any limitations or future directions for research.
- Conclusion : This section summarizes the main points of the research and provides a conclusion based on the findings. It may also suggest implications for future research or practical applications of the results.
- References : This section lists the sources cited in the research summary, following the appropriate citation style.
How to Write Research Summary
Here are the steps you can follow to write a research summary:
- Read the research article or study thoroughly: To write a summary, you must understand the research article or study you are summarizing. Therefore, read the article or study carefully to understand its purpose, research design, methodology, results, and conclusions.
- Identify the main points : Once you have read the research article or study, identify the main points, key findings, and research question. You can highlight or take notes of the essential points and findings to use as a reference when writing your summary.
- Write the introduction: Start your summary by introducing the research problem, research question, and purpose of the study. Briefly explain why the research is important and its significance.
- Summarize the methodology : In this section, summarize the research design, methods, and procedures used to conduct the study. Explain the sample size, data collection methods, and data analysis techniques.
- Present the results: Summarize the main findings of the study. Use tables, charts, or graphs to visually represent the data if necessary.
- Interpret the results: In this section, interpret the results and explain their implications. Discuss the significance of the findings, compare them to previous research, and identify any limitations or future directions for research.
- Conclude the summary : Summarize the main points of the research and provide a conclusion based on the findings. Suggest implications for future research or practical applications of the results.
- Revise and edit : Once you have written the summary, revise and edit it to ensure that it is clear, concise, and free of errors. Make sure that your summary accurately represents the research article or study.
- Add references: Include a list of references cited in the research summary, following the appropriate citation style.
Example of Research Summary
Here is an example of a research summary:
Title: The Effects of Yoga on Mental Health: A Meta-Analysis
Introduction: This meta-analysis examines the effects of yoga on mental health. The study aimed to investigate whether yoga practice can improve mental health outcomes such as anxiety, depression, stress, and quality of life.
Methodology : The study analyzed data from 14 randomized controlled trials that investigated the effects of yoga on mental health outcomes. The sample included a total of 862 participants. The yoga interventions varied in length and frequency, ranging from four to twelve weeks, with sessions lasting from 45 to 90 minutes.
Results : The meta-analysis found that yoga practice significantly improved mental health outcomes. Participants who practiced yoga showed a significant reduction in anxiety and depression symptoms, as well as stress levels. Quality of life also improved in those who practiced yoga.
Discussion : The findings of this study suggest that yoga can be an effective intervention for improving mental health outcomes. The study supports the growing body of evidence that suggests that yoga can have a positive impact on mental health. Limitations of the study include the variability of the yoga interventions, which may affect the generalizability of the findings.
Conclusion : Overall, the findings of this meta-analysis support the use of yoga as an effective intervention for improving mental health outcomes. Further research is needed to determine the optimal length and frequency of yoga interventions for different populations.
References :
- Cramer, H., Lauche, R., Langhorst, J., Dobos, G., & Berger, B. (2013). Yoga for depression: a systematic review and meta-analysis. Depression and anxiety, 30(11), 1068-1083.
- Khalsa, S. B. (2004). Yoga as a therapeutic intervention: a bibliometric analysis of published research studies. Indian journal of physiology and pharmacology, 48(3), 269-285.
- Ross, A., & Thomas, S. (2010). The health benefits of yoga and exercise: a review of comparison studies. The Journal of Alternative and Complementary Medicine, 16(1), 3-12.
Purpose of Research Summary
The purpose of a research summary is to provide a brief overview of a research project or study, including its main points, findings, and conclusions. The summary allows readers to quickly understand the essential aspects of the research without having to read the entire article or study.
Research summaries serve several purposes, including:
- Facilitating comprehension: A research summary allows readers to quickly understand the main points and findings of a research project or study without having to read the entire article or study. This makes it easier for readers to comprehend the research and its significance.
- Communicating research findings: Research summaries are often used to communicate research findings to a wider audience, such as policymakers, practitioners, or the general public. The summary presents the essential aspects of the research in a clear and concise manner, making it easier for non-experts to understand.
- Supporting decision-making: Research summaries can be used to support decision-making processes by providing a summary of the research evidence on a particular topic. This information can be used by policymakers or practitioners to make informed decisions about interventions, programs, or policies.
- Saving time: Research summaries save time for researchers, practitioners, policymakers, and other stakeholders who need to review multiple research studies. Rather than having to read the entire article or study, they can quickly review the summary to determine whether the research is relevant to their needs.
Characteristics of Research Summary
The following are some of the key characteristics of a research summary:
- Concise : A research summary should be brief and to the point, providing a clear and concise overview of the main points of the research.
- Objective : A research summary should be written in an objective tone, presenting the research findings without bias or personal opinion.
- Comprehensive : A research summary should cover all the essential aspects of the research, including the research question, methodology, results, and conclusions.
- Accurate : A research summary should accurately reflect the key findings and conclusions of the research.
- Clear and well-organized: A research summary should be easy to read and understand, with a clear structure and logical flow.
- Relevant : A research summary should focus on the most important and relevant aspects of the research, highlighting the key findings and their implications.
- Audience-specific: A research summary should be tailored to the intended audience, using language and terminology that is appropriate and accessible to the reader.
- Citations : A research summary should include citations to the original research articles or studies, allowing readers to access the full text of the research if desired.
When to write Research Summary
Here are some situations when it may be appropriate to write a research summary:
- Proposal stage: A research summary can be included in a research proposal to provide a brief overview of the research aims, objectives, methodology, and expected outcomes.
- Conference presentation: A research summary can be prepared for a conference presentation to summarize the main findings of a study or research project.
- Journal submission: Many academic journals require authors to submit a research summary along with their research article or study. The summary provides a brief overview of the study’s main points, findings, and conclusions and helps readers quickly understand the research.
- Funding application: A research summary can be included in a funding application to provide a brief summary of the research aims, objectives, and expected outcomes.
- Policy brief: A research summary can be prepared as a policy brief to communicate research findings to policymakers or stakeholders in a concise and accessible manner.
Advantages of Research Summary
Research summaries offer several advantages, including:
- Time-saving: A research summary saves time for readers who need to understand the key findings and conclusions of a research project quickly. Rather than reading the entire research article or study, readers can quickly review the summary to determine whether the research is relevant to their needs.
- Clarity and accessibility: A research summary provides a clear and accessible overview of the research project’s main points, making it easier for readers to understand the research without having to be experts in the field.
- Improved comprehension: A research summary helps readers comprehend the research by providing a brief and focused overview of the key findings and conclusions, making it easier to understand the research and its significance.
- Enhanced communication: Research summaries can be used to communicate research findings to a wider audience, such as policymakers, practitioners, or the general public, in a concise and accessible manner.
- Facilitated decision-making: Research summaries can support decision-making processes by providing a summary of the research evidence on a particular topic. Policymakers or practitioners can use this information to make informed decisions about interventions, programs, or policies.
- Increased dissemination: Research summaries can be easily shared and disseminated, allowing research findings to reach a wider audience.
Limitations of Research Summary
Limitations of the Research Summary are as follows:
- Limited scope: Research summaries provide a brief overview of the research project’s main points, findings, and conclusions, which can be limiting. They may not include all the details, nuances, and complexities of the research that readers may need to fully understand the study’s implications.
- Risk of oversimplification: Research summaries can be oversimplified, reducing the complexity of the research and potentially distorting the findings or conclusions.
- Lack of context: Research summaries may not provide sufficient context to fully understand the research findings, such as the research background, methodology, or limitations. This may lead to misunderstandings or misinterpretations of the research.
- Possible bias: Research summaries may be biased if they selectively emphasize certain findings or conclusions over others, potentially distorting the overall picture of the research.
- Format limitations: Research summaries may be constrained by the format or length requirements, making it challenging to fully convey the research’s main points, findings, and conclusions.
- Accessibility: Research summaries may not be accessible to all readers, particularly those with limited literacy skills, visual impairments, or language barriers.
About the author
Muhammad Hassan
Researcher, Academic Writer, Web developer
You may also like

Data Collection – Methods Types and Examples

Delimitations in Research – Types, Examples and...

Research Process – Steps, Examples and Tips

Research Design – Types, Methods and Examples

Institutional Review Board – Application Sample...

Evaluating Research – Process, Examples and...
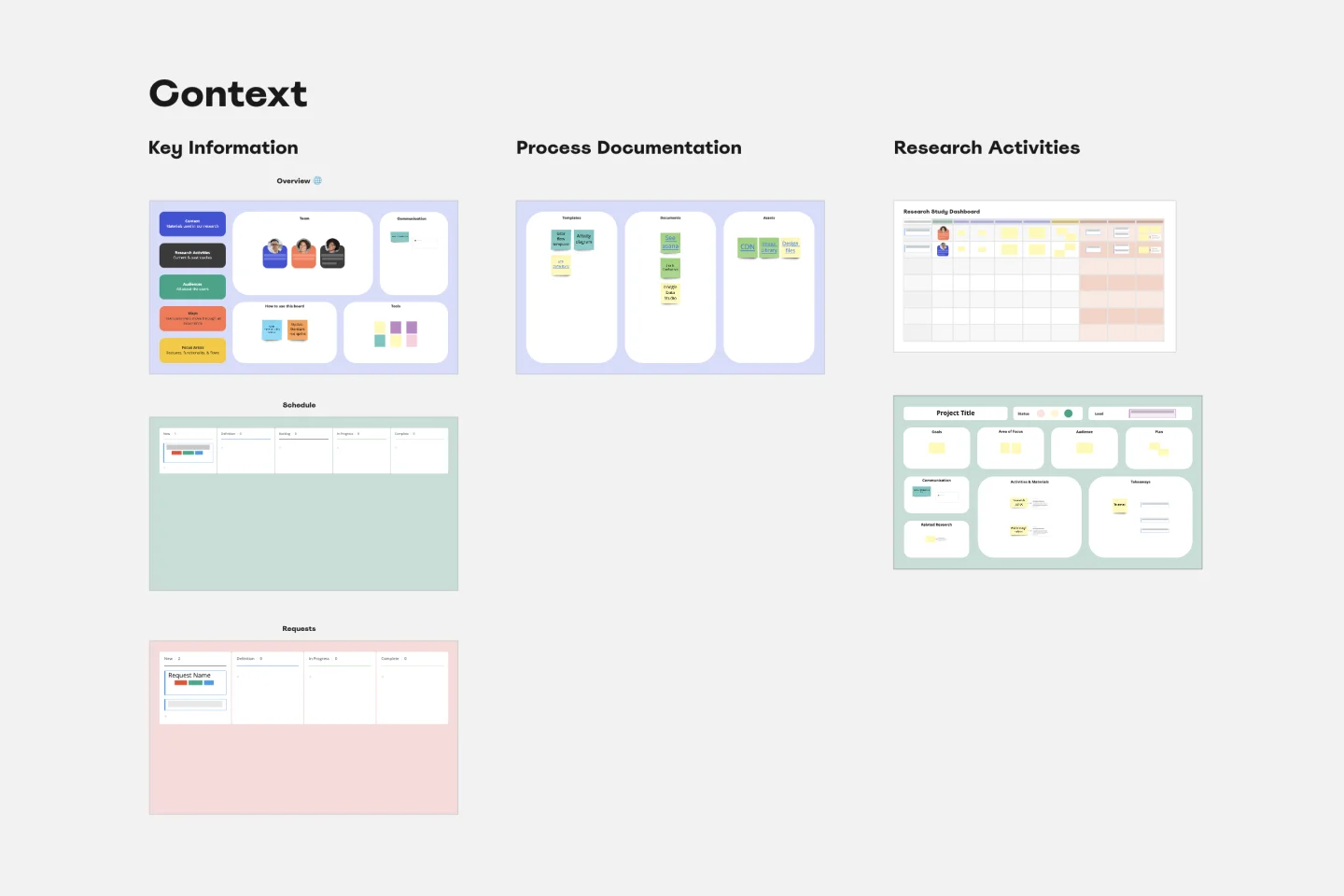
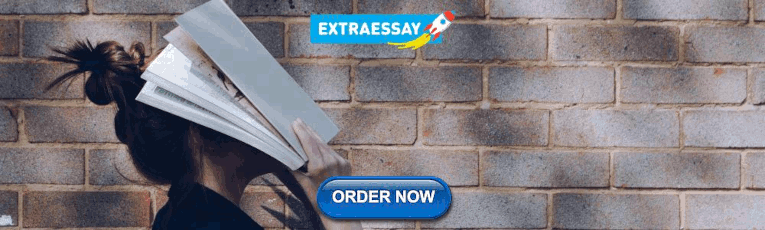
UX Research Repository Template
Empower your organization with customer knowledge and build a centralized research hub. From UX designers to product managers, enable everyone to get insights using the Research Repository Template.
Trusted by 65M+ users and leading companies
About the Research Repository Template
The Research Repository Template solves many problems around knowledge sharing and customer insights inside organizations. To break down silos between teams and build a healthy, human-centric company culture, UX researchers and designers came together and created the concept of a research hub. In this shared space, everyone can benefit from the insights and knowledge produced by UX research teams.
What is the Research Repository Template?
Put simply, the Research Repository Template contains all the information gleaned from user interviews. It serves as a database where you can look for insights and leverage decision-making. It’s also a great tool to educate your company on user research and enable teams to come up with innovative ideas based on the research findings.
The Research Repository Template also allows you to present your research with clarity, deliver insights faster and keep your report up to date, staying relevant for a longer time.
How everyone benefits from Research Repository Template
The Research Repository Template has many benefits. Above all, it helps create a company culture around research, where teams benefit from insights and make better-informed decisions. Here are a few of the pros of using repository research:
1. Connect teams around research
The Research Repository Template helps UX teams to share and advocate for UX research, connecting teams around research or even enabling them to do the research themselves, democratizing UX practices. It’s a win-win situation when research findings are used company-wide, you see strategies aligning, and results outperforming.
2. Enable teams to learn from insights
UX research teams can bring clarity to their research with a repository template and intuitively organize and track their findings. The repository in Miro acts as a database, and every team can consult and search for insights using the search bar (command+F) directly on the board. It’s also a visual way to organize your research data, providing a more guided experience for those not so familiar with UX insights.
3. Unify research across your organization
Put an end to slack pinging for data and insights! Have in one shared space your key research findings, helping to standardize and track all research in your organization, leveraging your research ops. Easily plan and conduct research across teams, and facilitate knowledge sharing with the Research Repository Template.
4. End silos
The research repository is a great way to bring awareness to leadership, involve important stakeholders in research, and give them access to valuable insights. Perfect for kickoffs, add to your project board a link to the Research Repository Template or share it with anyone leading a project that will benefit from customer insights.
Create a research hub
Setting up a research repository is not an easy task, and there are many ways of organizing and sharing research findings. Our product evangelist, Shipra Kayan, created this ready-made template keeping in mind the needs of UX research and other teams inside the organization.
The Research Repository Template allows you to organize all the information and materials related to your research in a visual, intuitive way, so that you can share it widely or consult it quickly, uncovering insights and opportunities.
The template is divided into two main sections:
Add all the project’s high-level information so UX teams and others can find research specifics inside your company. This section is divided into three sub-sections:
1. Key information
Here, you gather all the information UX teams or any other team might need to understand the research or actively participate in it.
Overview : Add info about the team and link from here to other parts of the research repository so people can easily access what interests them most.
Schedule : Use the schedule when something is in progress or if there is a problem with the research—track all projects in one shared space, to keep consistency and a great overview. To learn more details about a given project, click on the cards.
Requests : Use it as a ‘research backlog,’ giving the space for everyone to identify opportunities and start conversations around new research initiatives.
2. Process Documentation
Add any documents related to your research, including contracts and NDAs. This is a space where you can embed any file, links, and other resources you might find helpful to keep your team organized.
3. Completed Studies
This section of the research repository is where you share your research data. We advise you to use it when your research is almost complete, if not finished already. It’s where other teams can easily browse and look for what interests them.
Research study dashboard : Add high-level information, use cards to add more in-depth info, and assign them to the respective owners.
Projects : Present a summary of your research, allowing teams to view at a glance what the research is about or even add this frame to their presentations by copying and pasting it to other Miro boards.
Evergreen insights
Bring together your qualitative data, adding your personas and customer journeys. In this section, add cross-team insights and outline your focus areas and how your findings help you reach your goals.
This section is divided into:
Audiences: Add your user persona studies.
Maps: Add your customer journey maps.
Focus areas: Add your goals, related research, and steps to get there.
How do you organize users in research?
When leading or participating in user research, analyze all your UX-related frameworks and tools your team uses inside your organization. To organize users in research, opt for a framework that allows you to gather information in one shared space, so everyone in your team can access it. Consider trying using our Research Template and see if it works for you.
How do you keep track of user research?
Many teams use a research repository to keep track of user research. First, identify the inputs (everything related to planning and conducting research) and outputs (research findings and insights). When you create a research hub, it’s easier to track research findings and ongoing research, unifying your organization's UX research efforts. Use Miro’s Research Repository Template to help you build a research hub, allowing UX teams and others inside your organization to benefit from insights.
Get started with this template right now.
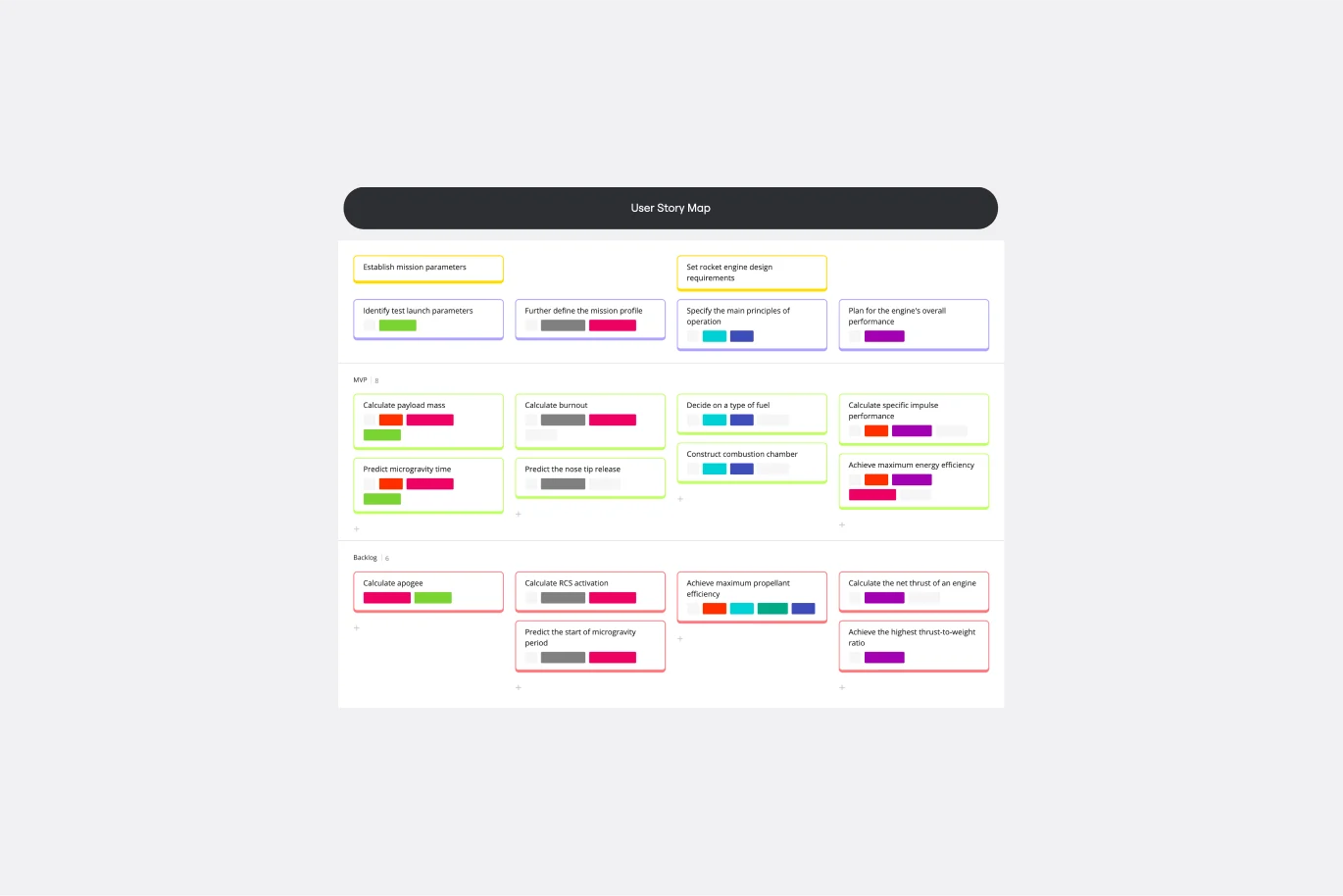
User Story Map Template
Works best for:.
Marketing, Desk Research, Mapping
Popularized by Jeff Patton in 2005, the user story mapping technique is an agile way to manage product backlogs. Whether you’re working alone or with a product team, you can leverage user story mapping to plan product releases. User story maps help teams stay focused on the business value and release features that customers care about. The framework helps to get a shared understanding for the cross-functional team of what needs to be done to satisfy customers' needs.
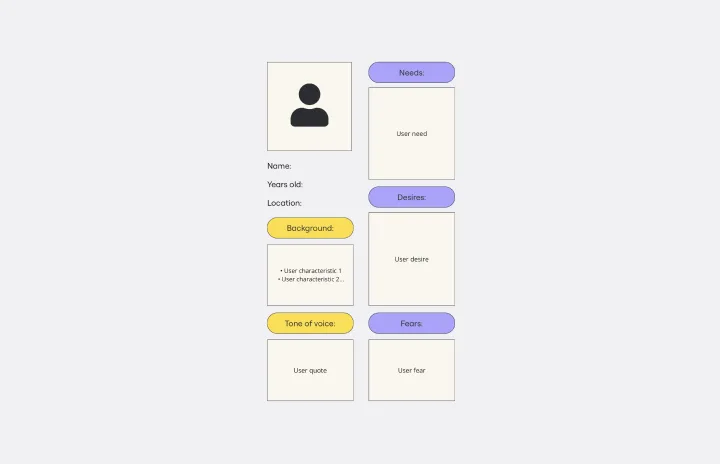
Proto Persona Template
UX, UX Research, Product Design
The Proto Persona Template is tailored to capture the essence of hypothetical user segments. It encapsulates key attributes such as user needs, behaviors, and potential pain points. One of its standout benefits is its ability to foster empathy. By visualizing and understanding these preliminary user profiles, design and strategy teams can tap into a deeper connection with their target audience, ensuring that solutions resonate authentically and address genuine needs.
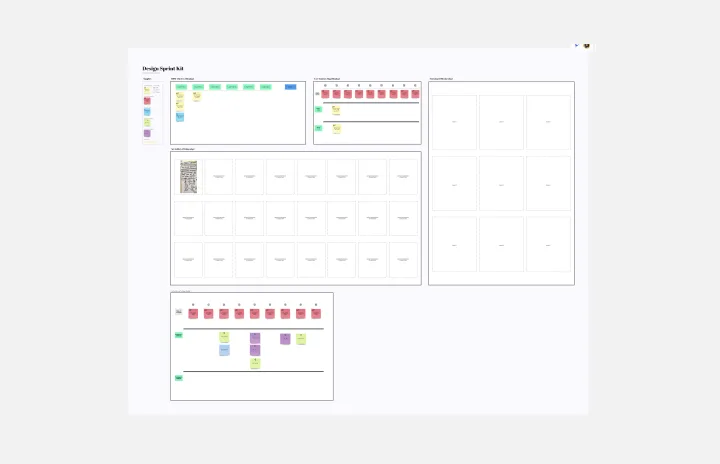
Design Sprint Kit Template
Agile Methodology, UX Design, Sprint Planning
With the right focused and strategic approach, five days is all it takes to address your biggest product challenges. That’s the thinking behind Design Sprint methodology. Created by Tanya Junell of Blue Label Labs, this Design Sprint Kit provides a set of lightweight templates that support the Design Sprint’s collaborative activities and voting—and maintains the energy, team spirit, and momentum that was sparked in the session. Virtual sprint supplies and prepared whiteboards make this kit especially useful for remote Design Sprint Facilitators.
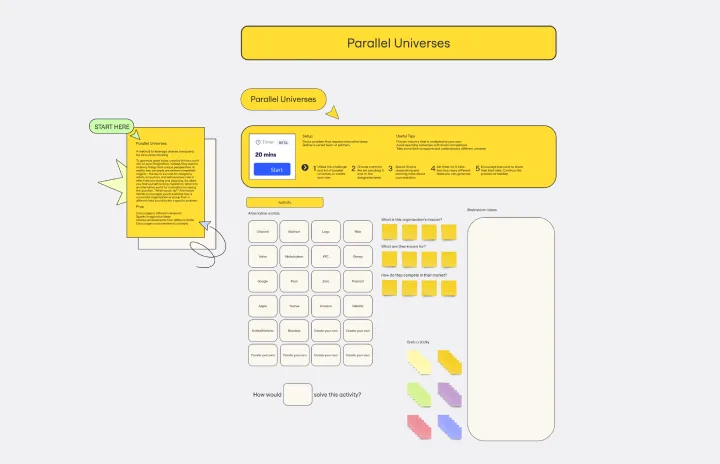
Parallel Universes Template
Creative ideas often come from exploring ordinary things from unique perspectives. The key to success is borrowing and adapting. The Parallel Universes Template encourages you to examine how a successful organization from a different field would tackle a specific problem.
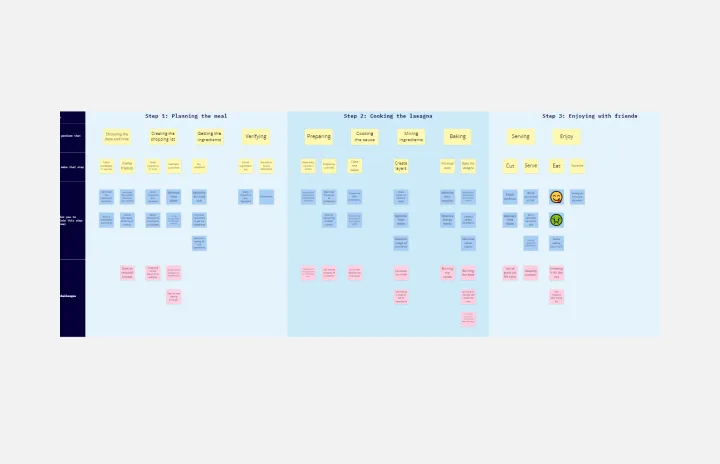
Job Map Template
Design, Desk Research, Mapping
Want to truly understand your consumers’ mindset? Take a look at things from their perspective — by identifying the “jobs” they need to accomplish and exploring what would make them “hire” or “fire” a product or service like yours. Ideal for UX researchers, job mapping is a staged process that gives you that POV by breaking the “jobs” down step by step, so you can ultimately offer something unique, useful, and different from your competitors. This template makes it easy to create a detailed, comprehensive job map.
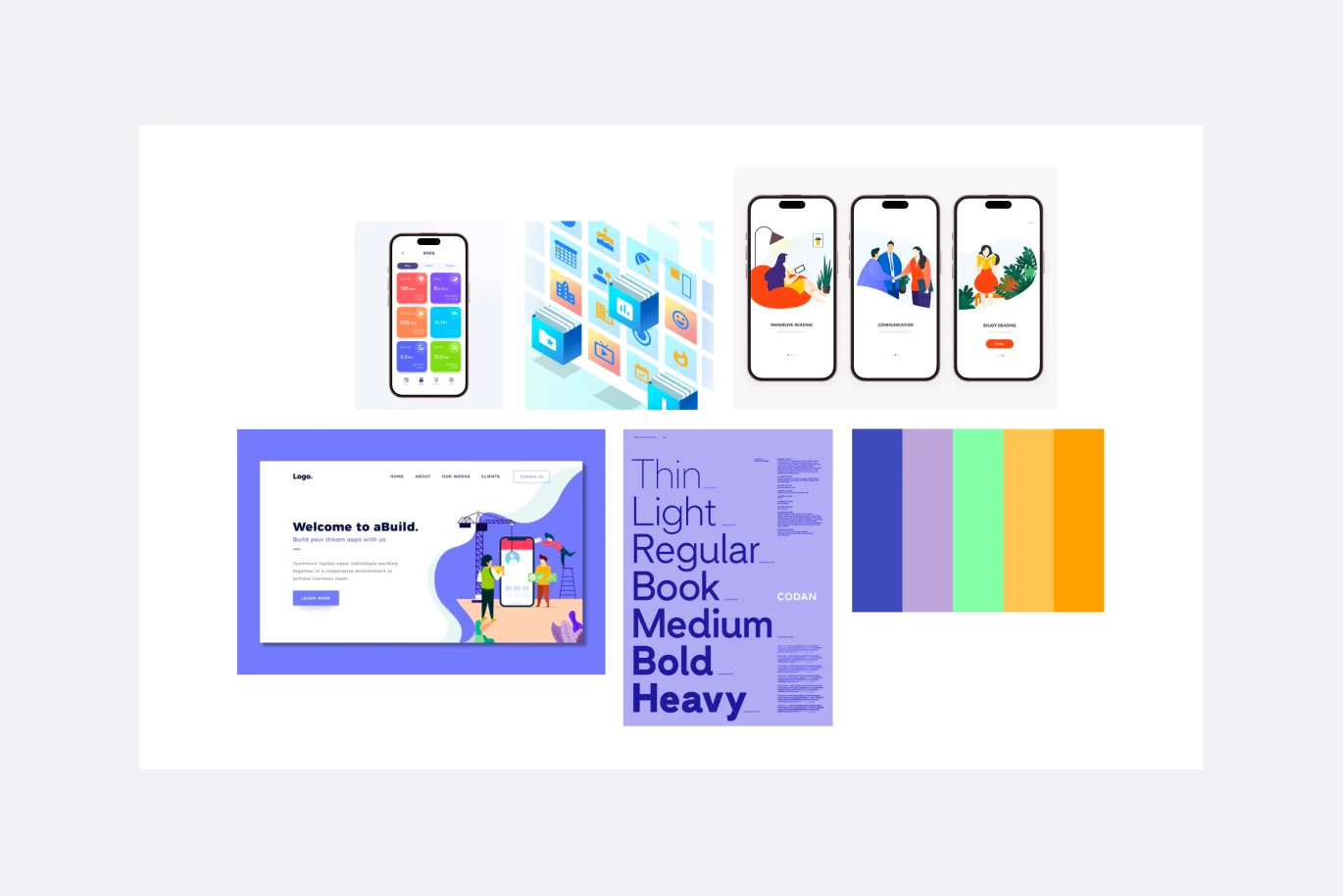
Mood Board Template
Design, Brainstorming, Ideation
When you’re kicking off a creative project, it’s sometimes important to communicate the mood you’re trying to evoke — but it’s so hard to do it with words. So create a mood board and use images, color palettes, textures, and typography. Mood boards are also perfect for gathering inspiration and sketching out and pitching ideas, and they’re not just for designers — your content writers, sales teams, and product teams can use them too, and this template makes it easy for all of you to get started.
- Open access
- Published: 15 May 2024
Alzheimer blood biomarkers: practical guidelines for study design, sample collection, processing, biobanking, measurement and result reporting
- Xuemei Zeng 1 ,
- Yijun Chen 2 ,
- Anuradha Sehrawat 1 ,
- Jihui Lee 1 ,
- Tara K. Lafferty 1 ,
- Julia Kofler 3 ,
- Sarah B. Berman 4 ,
- Robert A. Sweet 1 , 4 ,
- Dana L. Tudorascu 1 ,
- William E. Klunk 1 ,
- Milos D. Ikonomovic 1 , 4 , 5 ,
- Anna Pfister 6 , 7 ,
- Henrik Zetterberg 6 , 7 , 8 , 9 , 10 , 11 ,
- Beth E. Snitz 4 ,
- Anne D. Cohen 1 ,
- Victor L. Villemagne 1 ,
- Tharick A. Pascoal 1 , 4 ,
- M. llyas Kamboh 12 ,
- Oscar I. Lopez 4 ,
- Kaj Blennow 6 , 7 &
- Thomas K. Karikari ORCID: orcid.org/0000-0003-1422-4358 1 , 6
Molecular Neurodegeneration volume 19 , Article number: 40 ( 2024 ) Cite this article
1047 Accesses
32 Altmetric
Metrics details
Alzheimer’s disease (AD), the most common form of dementia, remains challenging to understand and treat despite decades of research and clinical investigation. This might be partly due to a lack of widely available and cost-effective modalities for diagnosis and prognosis. Recently, the blood-based AD biomarker field has seen significant progress driven by technological advances, mainly improved analytical sensitivity and precision of the assays and measurement platforms. Several blood-based biomarkers have shown high potential for accurately detecting AD pathophysiology. As a result, there has been considerable interest in applying these biomarkers for diagnosis and prognosis, as surrogate metrics to investigate the impact of various covariates on AD pathophysiology and to accelerate AD therapeutic trials and monitor treatment effects. However, the lack of standardization of how blood samples and collected, processed, stored analyzed and reported can affect the reproducibility of these biomarker measurements, potentially hindering progress toward their widespread use in clinical and research settings. To help address these issues, we provide fundamental guidelines developed according to recent research findings on the impact of sample handling on blood biomarker measurements. These guidelines cover important considerations including study design, blood collection, blood processing, biobanking, biomarker measurement, and result reporting. Furthermore, the proposed guidelines include best practices for appropriate blood handling procedures for genetic and ribonucleic acid analyses. While we focus on the key blood-based AD biomarkers for the AT(N) criteria (e.g., amyloid-beta [Aβ]40, Aβ42, Aβ42/40 ratio, total-tau, phosphorylated-tau, neurofilament light chain, brain-derived tau and glial fibrillary acidic protein), we anticipate that these guidelines will generally be applicable to other types of blood biomarkers. We also anticipate that these guidelines will assist investigators in planning and executing biomarker research, enabling harmonization of sample handling to improve comparability across studies.
Alzheimer’s disease (AD), the most common form of dementia, poses significant economic and social burden on affected individuals, as well as their families, caregivers, communities, and healthcare systems worldwide [ 1 ]. An estimated excess of 50 million people are living with AD globally, including 6.7 million in the United States. By 2050, these numbers are expected to rise to 152 million and 13.8 million, respectively [ 2 , 3 ]. Neuropathologically, AD is characterized by two hallmark lesions in the brain; amyloid-β (Aβ) plaques and tau neurofibrillary tangles [ 4 , 5 ]. Most individuals with brain pathophysiological evidence of AD clinically show progressive cognitive impairment [ 6 ]. Unfortunately, despite decades of research and numerous clinical trials, AD remains difficult to treat, with only a few FDA-approved drugs available for treatment [ 1 ]. Among them, lecanemab, donanemab and aducanumab, humanized antibodies designed to reduce the amyloid plaque burden, are the only ones expected to provide disease-modifying therapy [ 7 , 8 , 9 ]. The other drugs are palliative treatments that reduce the symptoms temporarily but are not directed toward preventing or slowing disease progression.
The slow pace of AD drug development is partly due to a lack of accessible and cost-effective biomarkers for participant enrollment and stratification in clinical trials. The National Institute on Aging and the Alzheimer’s Association (NIA-AA) research framework recommends the use of biomarker criteria for amyloid pathology, tau pathology, and neurodegeneration [AT(N)] for a biological definition of AD [ 10 ]. However, these assessments are currently performed using expensive, time-consuming, and sometimes invasive procedures with limited global accessibility such as magnetic resonance imaging (MRI), positron emission tomography (PET) scans, and/or cerebrospinal fluid (CSF) biomarkers [ 11 , 12 , 13 ], which are unsuitable for large-scale clinical applications and population screenings. It has been estimated that screening a single participant for AD clinical trials with PET and MRI could cost at least US $8,000 [ 14 ]. Given the typically high screen-failure rate (percentage of screened participants not meeting the enrollment criteria), it is not surprising that participant screening may cost 50–70% of total per-participant costs [ 14 ]. These costs could be prohibitive for large-scale clinical trials. In terms of clinical applications, imaging all patients with suspected cognitive impairment due to AD using PET and MRI would be difficult to achieve due to the low throughput and the limited availability of the specialized facilities and expertise needed to administer and interpret these tests [ 13 ].
To address this issue, there is a growing need to develop less invasive, more cost-effective, and scalable biomarkers that can reliably identify AD pathology. Blood-based AD biomarkers are a desirable choice due to availability of blood specimens through routine clinical practice and research programs. In clinical trials, blood biomarkers have already shown utility as pre-screening measures to streamline the identification and inclusion of individuals who fit pre-defined criteria for biological evidence of disease [ 15 , 16 ]. Importantly, clinical prescriptions of the recent FDA-approved anti-amyloid drugs require prior confirmation of brain amyloidosis. However, since amyloid PET and CSF Aβ42/Aβ 40 assessments are not feasible in many hospital settings, blood biomarkers would be very useful proxies.
The development of such biomarkers has been hindered by the extreme complexity of the blood proteome, low biomarker abundance, and signal dilution from peripheral tissues. However, significant advances have been achieved in the past decade, benefiting partly from the development of ultra-sensitive immunoassays and high-performance mass spectrometry technology platforms [ 17 , 18 , 19 , 20 ]. Blood-based biomarkers with high potential of providing accurate assessment of the AT(N) criteria include the Aβ42/40 ratio for amyloid pathology, phosphorylated tau (p-tau) for tau pathology, and neurofilament light-chain (NfL) and brain-derived tau for neurodegeneration/axonal injury [ 11 , 21 ]. In addition, plasma glial fibrillary acidic protein (GFAP), an indicator of reactive astrogliosis often associated with brain Aβ plaques, has also been proposed as an early marker for amyloid pathology [ 22 , 23 , 24 , 25 ].
The anticipated next stage in the development of highly sensitive and specific blood biomarkers for AD is to employ them in real-world settings for clinical diagnosis, population studies, and eligibility screening for therapeutic trials. However, a major challenge facing the field is the need for increased standardization of collection, processing, and storage procedures. Another important challenge is the need for agreed-upon procedures to monitor and maintain long-term stability in the biomarker measurements, especially since none of the blood-based AD biomarkers in use currently has certified reference measurement procedures. These obstacles must be overcome before blood-based biomarkers can be effectively adopted in clinical and research-based settings, and these measurements can be appropriately harmonized. A survey of studies across fifteen centers revealed variations in sample processing, such as the time of day for collection, fasting status, time from collection to centrifugation, the temperature at various steps, and centrifugation parameters [ 26 ]. The lack of standardization can introduce measurement variations, reducing clinical reliability and making it challenging to compare results across laboratories and establish clinical thresholds. These variations can be introduced at three phases: preanalytical, analytical, and post-analytical. The advancement of analytical technologies, such as automation in sample preparation, has dramatically reduced errors in the analytical phase. It is now thought that the preanalytical stage is the most error-prone phase (over 60%), followed by the post-analytical phase (over 20%) [ 27 ].
Decades of research have led to the development of a consensus protocol for standardized collection and biobanking of CSF-based AD biomarkers [ 28 ], which has been key to the much improved inter-laboratory reproducibility of the core CSF biomarkers in recent years [ 29 ]. Blood-based AD biomarkers often have smaller effect sizes than the corresponding CSF biomarkers (with the exception of GFAP) [ 25 ], possibly due to signal attenuation caused by counterpart proteins secreted or biomarkers sequestered by peripheral tissues and the increased biological complexity of blood [ 13 , 30 ] (further discussed below). Therefore, minimizing preanalytical variations is even more critical for blood-based AD biomarkers. O’Bryant et al., in 2015, proposed a set of guidelines to standardize blood sample collection [ 31 ]. Yet, the evidence evaluating defined preanalytical factors was limited at that time. Since then, many research studies have been published, and an updated evidence-based plasma handling standardized operating procedure (SOP) was proposed in 2022 by Verberk et al. [ 26 ]. However, this SOP was limited to preanalytical factors and was directed at experienced blood biomarker laboratories and scientists. As blood biomarkers become more widely available and simplified commercial technologies get increasingly accessible, fundamental guidelines that: (1) take into account the preanalytical, analytical, and post-analytical pipeline; (2) accommodate the needs of investigators new to the blood biomarker space; and (3) are applicable to both traditional (blood collection and assessments in a clinical setting) and non-traditional (home collections, population-based evaluations, resource-limited settings) environments, are needed.
Standardization of blood AD biomarker research across sites, studies and investigators must consider several steps, including study design, blood collection, blood processing, biobanking, biomarker measurement, and result reporting (Fig. 1 ). This review aims to expand on earlier guidelines by employing the evidence base from recent research findings and our own experiences to provide a detailed description of the general considerations associated with each of these steps. Furthermore, we present an easy-to-follow SOP to aid in the design and implementation of high-quality blood-based AD biomarker research projects, covering the preanalytical, analytical, and post-analytical phases. It is worth noting that although we focus on the key blood-based AD biomarkers for the AT(N) criteria (e.g., Aβ40, Aβ42, Aβ42/40 ratio, total-tau (t-tau), p-tau, NfL, brain-derived tau [BD-tau] and GFAP), we anticipate that these guidelines will generally be applicable to other types of blood biomarkers as well as discovery proteomic investigations.
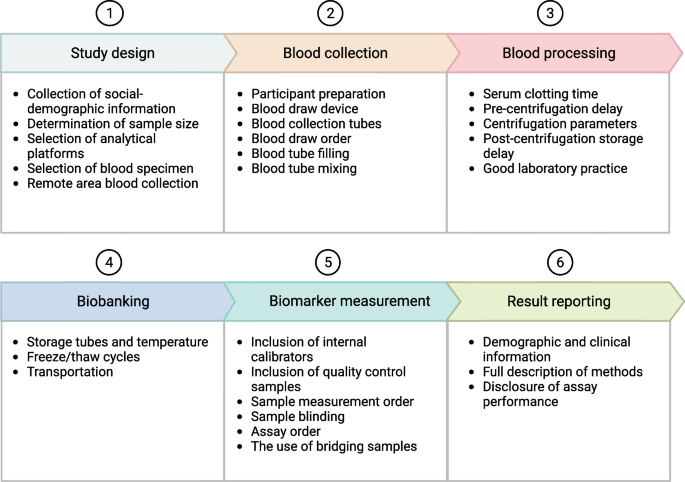
Proposed general workflow for conducting high-quality blood AD biomarker research and the important considerations associated with each step. The major steps are divided into six, namely study design, blood collection, blood processing, biobanking, biomarker measurement and results reporting. The important considerations under each step are listed in the Figure. Detailed discussions are provided in the text
Considerations during study design
Item 1: collection of sociodemographic, lifestyle, and health information.
Numerous sociodemographic, lifestyle, health, and environmental factors have been associated with an increased risk of AD [ 32 , 33 , 34 ]. For example, age, education, sex, race, creatinine levels, concomitant medication (including anti-amyloid therapies), medical history, and comorbidities such as diabetes, hypertension, impaired kidney function, liver diseases, and cardiovascular diseases have been found to be significantly associated with blood AD biomarkers levels in some research cohorts compared with unaffected controls [ 7 , 35 , 36 , 37 , 38 , 39 , 40 , 41 , 42 , 43 ]. Additionally, conditions such as pregnancy, menopausal symptoms and hormone therapy use are critical as biomarker changes have been reported [ 44 , 45 ] but require further investigation. While it may not be feasible to include all demographic and clinical information, we recommend recording as much information as possible during subject recruitment and through the medical record extraction to prevent over-/under-interpretation of results and to enable adjustment of reference ranges according to population characteristics if needed. Importantly, the collection, processing, storage, and application of personal data must conform with the prevailing ethical guidelines and legal frameworks in the countries concerned. For example, there is the General Data Protection Regulation (GDPR) in Europe and Health Insurance Portability and Accountability Act of 1996 (HIPAA) in the United States.
Item 2: sample size consideration
Sample size planning is critical in studies evaluating the diagnostic and prognostic capabilities of blood AD biomarkers and the impact of covariates. Insufficient sample size reduces the power to detect true significance. In contrast, excess sample size may magnify the importance of clinically non-meaningful differences, increase costs, prolong the study, and potentially expose more participants to needless evaluations [ 46 ]. Blood AD biomarkers often have smaller effect sizes than CSF biomarkers due to the expected dilution effect from peripheral tissues. Aβ42/40 ratio, for example, has reduced difference between Aβ+ vs. Aβ- individuals from ~ 40% in CSF to ~ 10% in blood [ 13 , 25 , 30 ]. Similarly, p-tau fold change is comparatively decreased from 166% in CSF to 85.6% in the blood [ 13 ], despite plasma p-tau having larger effect sizes than plasma Aβ42/40 ratio when compared directly [ 25 , 47 ]. Therefore, blood AD biomarker studies will require larger sample sizes than CSF biomarker studies. To determine the optimal sample size, one must consider factors such as expected effect size, population variance, desirable type I and type II error rates, participant dropout rate for longitudinal studies, and adjustment of covariates [ 46 ]. It is important to note that sample size calculation can become challenging for complex studies and will require statisticians’ advice during study design.
Item 3: selection of analytical platforms
Blood AD biomarkers tend to be near or below the detection limit of traditional enzyme-linked immunosorbent assays (ELISAs). However, in the past decade, many technologies have emerged that have significantly accelerated research in this area [ 18 , 19 , 48 , 49 , 50 , 51 , 52 , 53 , 54 , 55 , 56 , 57 , 58 , 59 , 60 , 61 , 62 ]. Among them, Single molecule array (Simoa), mass spectrometry, as well as immunoassay technologies available on platforms such as the Elecsys and Cobas systems from Roche Diagnostics, Meso Scale Discovery (MSD), and immunomagnetic reduction (MagQu) are the most used. Recently, the Lumipulse G system, widely used for running electrochemiluminescence (ECL) immunoassays in CSF samples for research and in vitro diagnostic purposes, has also moved into the blood biomarker space, with assays now available for plasma Aβ peptides, NfL, p-tau181 and p-tau217 [ 63 ]. In addition, Ella, a novel platform for running multi-analyte automated microfluidic immunoassays (Simple Plex™) has now entered the AD biomarker field, with assays available for plasma NfL [ 64 , 65 ]. Emerging platforms include Nano Mosaic and NULISA. We have summarized in Table 1 the commonly used platforms for the key AD blood biomarkers, but the list is not meant to be exhaustive. For more detailed descriptions, readers should refer to recent review articles [ 20 , 66 , 67 , 68 ] and references therein.
Extensive studies have been conducted to examine associations of blood biomarkers with AD pathology and compare the performance of different platforms. While a comprehensive review of the literature is beyond the scope of this work, a brief summary of the key findings is provided below:
Amyloid plaques, one of the primary pathological features of AD, consist mainly of amyloid beta peptides [ 77 , 78 ]. While CSF Aβ42/40 has been used in clinical settings to assess brain Aβ plaques, the association of blood Aβ42/40 with AD pathologies has been controversial [ 79 , 80 ]. Several immunoassays and MS assays are available to measure blood Aβ peptides [ 20 ], but overall, there is low inter-platform reproducibility [ 75 , 81 ]. MS assays generally exhibit superior predictive power for brain Aβ compared to immunoassays, possibly due to higher specificity obtained through MS assays [ 81 ].
CSF t-tau is a biomarker for neurodegeneration or neuronal injury [ 82 ]. However, plasma t-tau shows low correlation with CSF t-tau due to potential contamination with tau from peripheral sources [ 83 , 84 ]. Improved plasma t-tau assays have been reported recently [ 85 , 86 ]. In addition, recently developed Simoa assay targeting brain-derived tau showed a better correlation with CSF t-tau and improved biomarker performance [ 21 ].
CSF p-tau is a biomarker for neurofibrillary tangles [ 15 , 87 , 88 ]. Despite their low abundance in the blood, several assays are available to measure p-tau species in the blood [ 13 , 89 , 90 , 91 , 92 ]. Unlike plasma Aβ assays, p-tau assays exhibited overall strong inter-platform concordance [ 20 , 93 , 94 , 95 , 96 ]. P-tau181, p-tau217, and p-tau231 are the most widely studied p-tau species. P-tau212 is a new marker recently reported [ 97 ]. Different p-tau species might increase at different stages of the AD continuum [ 91 , 98 ]. Unlike their CSF counterparts, blood p-tau exhibits better association with Aβ plaques rather than neurofibrillary tangles.
GFAP is a biomarker for reactive astrogliosis [ 99 ], a cellular response often associated with brain Aβ plaque pathology in AD [ 100 ]. Plasma GFAP positively correlated with Aβ burden and tau pathology in AD [ 101 , 102 ]. Plasma GFAP level may be impacted by non-AD brain injuries and is an FDA-approved biomarker for detecting intracranial lesions after brain injury [ 103 ].
Neuronal damage/injury leads to elevated secretion of NfL into the extracellular space [ 104 ]. Although non-AD specific, NfL is an excellent biomarker for neurodegeneration to monitor the disease progression of AD patients [ 105 , 106 ]. Head-to-head comparison of Simoa and Ella assays in a multiple sclerosis cohort demonstrated a strong correlation between the platforms [ 64 , 65 ]. Plasma/serum brain-derived tau showed stronger specificity to AD pathophysiology versus related non-AD disorders.
Item 4: selection of blood specimen
Both plasma and serum have been utilized for measuring AD biomarkers. Studies comparing AD biomarker levels in serum and plasma have shown that some analytes, including Aβ peptides, t-tau, BD-tau and multiple p-tau species, are present at lower levels in serum, possibly due to a loss from clot trapping [ 26 , 107 , 108 , 109 , 110 , 111 ]. This makes it more challenging to measure such biomarkers in serum, especially for individuals whose biomarker levels are close to the lower detection limit. Nonetheless, biomarkers such as p-tau231, p-tau181 and BD-tau have been shown to have equivalent diagnostic accuracies in plasma and serum [ 21 , 89 , 107 , 109 , 111 ]. P-tau217 [ 112 ] and p-tau212 [ 97 ] are currently measurable in plasma but not serum. It is important to note that the choice of the blood specimen depends on the overall research objectives and sample availability. For example, serum may be a better choice for studies that evaluate the integrity of the blood-brain barrier since the CSF/serum albumin ratio is a well-established indicator of blood-CSF barrier function [ 113 ]. Additionally, serum is more widely used in hospital systems, with more clinical tests using serum instead of plasma as the specimen, according to the Mayo Clinic 2023 Test Catalog [ 114 ].
On the other hand, many research cohorts collect plasma instead of serum. Ethylenediaminetetraacetic acid (EDTA), heparin, and citrate are the most commonly used anticoagulants in clinics for plasma collection [ 115 ]. EDTA is the most universal in AD biomarker research [ 26 ]. Several studies have suggested that citrate plasma has lower levels of several biomarkers, including Aβ peptides, NfL, GFAP, and t-tau, compared with EDTA and heparin plasma [ 116 , 117 ]. However, studies comparing heparin vs. EDTA have generated mixed results. For example, one study found most biomarkers to be more abundant in heparin plasma than EDTA plasma [ 107 ], while another found higher levels of t-tau and p-tau181 but similar levels of Aβ40 and Aβ42 in heparin compared with EDTA plasma samples [ 118 ]. Rózga et al., on the other hand, reported that the levels of t-tau were significantly lower in heparin plasma [ 116 ].
Regardless of the type of blood specimen chosen, it is important to use the same type of specimen throughout the study. Although biomarkers may show a similar trend in different specimens, they are not necessarily linearly correlated in samples from all individuals. For example, despite strong correlations and similar diagnostic accuracy between paired serum and plasma p-tau levels, Kac et al. observed larger disagreements in samples with lower p-tau concentrations [ 109 ].
Item 5: blood collection from remote areas, under-resourced settings, or home care
Advanced laboratory equipment, such as ultra-low temperature freezers and centrifuges, are typically required for the processing of traditional venipuncture-based blood specimen. This can create significant obstacles for community-based studies utilizing home sampling, as well as for studies in remote areas, where access to such equipment may be limited. In addition, venipuncture may be difficult and painful for individuals with small or fragile veins [ 119 ]. To overcome these challenges, some research initiatives have explored alternative blood collection methods. For instance, Walter et al. compared Aβ40 and Aβ42 levels in conventional venous blood vs. capillary blood collected by finger insertion using microvettes. They found a good correlation between the two specimen types, despite slightly lower levels in capillary blood [ 120 ]. Similarly, Lombardi et al. and Simrén et al. investigated the use of dried blood spots (DBS) and dried plasma spots (DPS) for NfL measurement and found that NfL levels in both DBS and DPS samples correlated strongly with those in EDTA plasma samples with a stronger correlation observed for DPS samples [ 121 , 122 ].
Considerations during blood collection
Item 1: preparation of participants for the blood draw.
Studies examining the impact of pre-blood draw activities of participants, such as fasting, physical exercise, medication use, and the time of day for blood collection, are limited. However, preliminary evidence shows that some of these factors can impact blood biomarker levels. For example, Rózga et al. found that the levels of Aβ40 and Aβ42 were 5–9% higher in blood samples collected in the afternoon compared with those collected in the morning, with the opposite trend noted for t-tau [ 116 ]. Meyer et al. found significantly higher Aβ40 and Aβ42 levels in non-fasting blood when measured with Simoa assays [ 123 ]. Signal variation from plasma samples obtained on consecutive days from the same individual or within a cohort, which allows for the evaluation of short term fluctuation, have also been reported [ 124 ].
To minimize potential bias arising from these effects, we recommend pre-defining the participant preparation protocol upfront and following it throughout the study. Ideally, blood should be drawn at the same time of the day for all participants with the same fasting status throughout the study. If practical in the population under study, fasting blood samples may be more reliable in general. It is also important to record information such as blood draw time, fasting status, date and time of last meal, hours of sleep the previous night, pre-blood draw exercise activity, and medication use, to facilitate downstream interpretation of results.
Item 2: blood draw devices
Blood can be collected from participants in various ways, including venipuncture using an evacuated system (Vacutainer®), a syringe, butterfly needles, intravenous (IV) catheters, fingersticks, or heelsticks. The choice of blood draw methods depends on factors such as patient characteristics, the type of tests to be performed, and the preference and experience of clinical staff. Venipuncture using a needle and vacutainer tubes are the most used for routine blood draw. It is important to note that the devices used for the blood draw may influence the blood sample quality. For example, IV catheters and smaller-bore needles are sometimes used for patients with hard-to-access veins or when multiple blood draws over an extended period are needed. However, both have been found to have a higher hemolysis rate [ 125 , 126 ]. Additionally, lubricant coating and needle material, if released into the blood, can potentially contaminate the specimens, which have been shown to affect antigen-antibody binding in some immunoassays [ 127 ]. There is a lack of published research on the impact of blood draw devices on blood AD biomarker levels, aside from the type of blood collection tubes used (described below). To harmonize the blood collection procedures across different labs, O’Bryant et al. recommended using 21-gauge needles for blood draw in adults [ 31 ]. When possible, new straight needle venipuncture is preferred over the IV start. Any variation from standard procedures should be carefully documented.
Item 3: blood collection tubes
Most biomarker studies use evacuated tube systems for blood collection. There are many different brands of evacuated tube systems available, including BD’s Vacutainer®, Sarstedt’s Monovette®, and Greiner Bio-One’s VACUETTE®. Different blood collection tubes vary in materials, shape, size, additives used, and safety features. The color of the tube closure typically indicates the additives. We have listed some commonly used blood collection tubes and their intended clinical applications in Fig. 2 . Various components of the evacuated tubes, such as their surface coating, stopper materials, stopper lubricants, gel separators, and additives, can interfere with clinical laboratory assays and are potential sources for preanalytical variation [ 115 , 127 , 128 ]. For instance, gel separators – inert gels used as barriers for better separation of serum or plasma from cells/clots after centrifugation – have been shown to absorb blood constituents and interfere with various clinical tests for therapeutic drug monitoring [ 129 ]. It is worth noting that several studies indicated that AD biomarkers, especially Aβ peptides in the CSF matrix, may exhibit varying absorption rates to tube walls based on the materials they are made of. Specifically, there is a higher overall loss when polystyrene tubes are utilized [ 130 , 131 , 132 ]. Pre-treating the tubes with detergent Tween-20 might mitigate the absorption [ 133 , 134 ]. However, currently, there is no evidence to suggest that the primary materials used in blood tubes have any effect on AD biomarker levels [ 116 , 135 ]. To reduce the risk of variability caused by using different types of tubes, we suggest using the same brand of tubes consistently throughout the studies and limiting the number of lots to as few as possible.
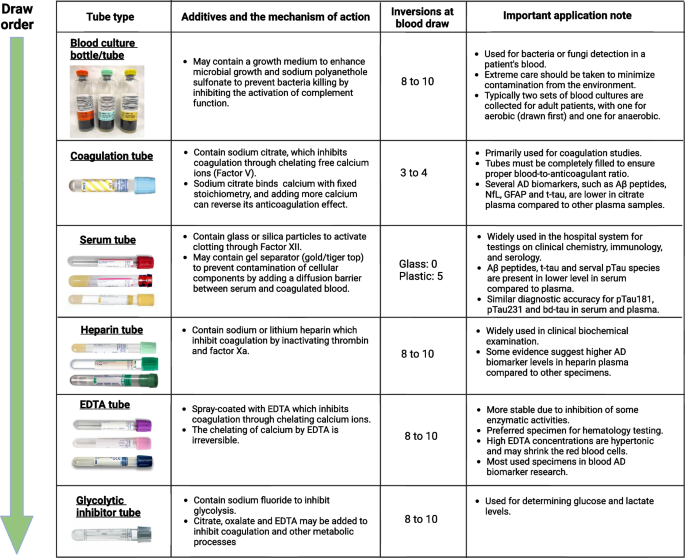
Commonly used human blood collection tubes, their draw order, additives, and important application notes. The numbers of inversions are based on BD’s recommendations for Vacutainer® tubes
Item 4: blood draw order
Different blood collection tubes contain distinctive additives. To minimize the impact of additive cross-contamination when collecting samples from a single blood draw into different types of tubes, the Clinical and Laboratory Standards Institute [ 136 ] CLSI; https://clsi.org/ ) recommended a specific blood draw order (as shown in Fig. 2 ): (1) blood culture tube or bottle; (2) sodium citrate tube; (3) serum tube; (4) heparin tube; (5) EDTA tube; (6) sodium fluoride/potassium oxalate glycolytic inhibitor tube. Following the recommended blood draw order is crucial to avoid carry-over additives that may result in inaccurate results. For example, if an EDTA tube is drawn before a serum tube, some of the EDTA may carry over into the serum tube and interfere with the coagulation.
Item 5: blood collection tube filling height
Some collection tubes, such as those for plasma collection, contain spray-coated or liquid additives. Therefore, different tube filling heights may cause variations in the blood-to-additive ratio, potentially influencing the protein composition. In support of this, Rózga et al. found a lower level of plasma t-tau when K 2 -EDTA tubes were filled only to 50% compared to 100% [ 116 ]. Therefore, to minimize variation, we recommend adhering to manufacturers’ recommendations for blood volume filling to maintain a consistent additive-to-blood ratio among all samples.
Item 6: proper mixing of blood samples
For blood collection tubes containing additives, it is crucial to gently invert the tubes immediately after the blood draw to ensure proper mixing of the additive with the blood. Failure to do so may result in non-homogenous samples and the formation of microclots or residual fibrins that can obstruct the sample probe of analytical instruments. The number of required inversions varies by tube type. We suggest following the manufacturer’s guidelines for the mixing. The recommended number of inversions for BD’s Vacutainer® tubes is listed in Fig. 2 .
Considerations during blood processing
Both serum and plasma are liquid components derived from blood after separating the blood cells, typically through centrifugation. The main difference is that serum is collected from clotted blood, while plasma is collected without clotting through anti-coagulants, thus retaining the clotting factors. Apart from the variations mentioned above for blood collection, several factors during blood processing, including pre-centrifugation delay time, centrifugation conditions, post-centrifugation storage delay, and temperature at various processing steps, may also contribute to the pre-analytical variation of the resulting blood specimens. In the following sections, we summarize current research findings on the impact of these variables and provide general guidelines for blood processing. Additionally, we have included a detailed step-by-step SOP for collecting plasma from EDTA tubes in Additional file 1 .
Item 1: serum clotting time
Harmonizing SOPs for serum collection can be challenging, partly because of the difficulty in setting the optimal clotting time. Insufficient clotting may lead to the formation of residual fibrin, which may clog the biomarker-measuring instruments [ 137 ]. In contrast, prolonged clotting may lead to cell lysis, resulting in serum contamination with cellular components [ 138 ]. The ideal clotting time varies not only by tube type but also by patient characteristics. Plain red top tubes (glass with no additive or plastic coated with silica as clot activator) and serum separator tubes (SST; gold top or tiger top) with clot activators and gel separators are commonly used in the clinics for serum collection. BD Diagnostic recommends a 30-min clotting time for SST and a 60-min clotting time for the red top tubes. Patients with certain diseases, such as liver diseases and multiple myeloma, or those on anticoagulant therapy, may require a longer clotting time. It has therefore been recommended that blood samples should be left to sit upright at room temperature for at least 30 min but no more than 60 min to allow clots to form and minimize the interference of blood cell lysis [ 31 ].
Item 2: pre-centrifugation delay time
Several studies have investigated the impact of pre-centrifugation delay on AD blood biomarker measurements. A long delay has been associated with a more significant decrease in biomarker levels. This impact can be mitigated by storing blood at 4 °C rather than room temperature prior to centrifugation [ 26 , 116 , 120 ]. Among the AD blood biomarkers, Aβ peptides are particularly susceptible to loss from pre-centrifugation delay. Their levels drop in a time-dependent manner when stored at room temperature [ 139 ]. To minimize interference of blood cell lysis and protein degradation, it is recommended completing the whole process within 2 h [ 31 ] and, if not feasible, keeping the blood refrigerated for no more than 24 h [ 26 ]. However, the shorter the pre-centrifugation delay, the better for all the blood biomarkers.
Item 3: centrifugation settings, including speed, time, and temperature
Optimal centrifugation settings are crucial for obtaining high-quality serum/plasma samples. Prolonged or excessive-speed centrifugation may cause blood cell lysis, while centrifugation that is too short and/or at an insufficient speed may result in incomplete separation of serum/plasma from blood cells [ 140 ]. Centrifugation settings may vary by blood collection tube type. For example, coagulated tubes require longer centrifugation than plasma tubes to ensure complete serum separation from the clot (CLSI H21). According to a recent survey [ 26 ] the common practice in the blood AD biomarker field is to centrifuge for 5–15 min at 1500–3000 xg. However, there are still very limited studies evaluating the impact of centrifugation parameters on AD blood biomarker measurements. A preliminary investigation found no significant difference between room temperature and 4 °C centrifugation for most AD biomarkers except t-tau, whose abundance was lower with 4 °C centrifugation [ 26 ].
Item 4: post-centrifugation storage delay
Post-centrifugation delay may also contribute to a decrease in biomarker abundance, although the rate of decline appears slower than during pre-centrifugation delay [ 26 , 120 ]. Similarly, keeping samples on wet ice while waiting for storage has been found to greatly mitigate the impact of storage delay.
Item 5: good laboratory practice (GLP)
Adhering to GLP during blood processing is crucial for ensuring the safety, quality, and integrity of research studies. Below are some key practices that should be followed:
All blood samples and associated collection devices should be considered potentially infectious, and proper personal protective equipment (PPE) should always be used to minimize exposure risk.
To protect the confidentiality of research participants, personal information should not be included on specimen labels. To avoid sample mix-up, all tubes should be clearly labeled, preferably using printed labels or barcodes rather than handwritten ones. This labeling should be done in advance of the participants’ visits for blood collection.
Good pipetting skills are essential for ensuring high sample quality. When pipetting plasma/serum from the blood collection tubes, gently draw the liquid from the top and gradually move the pipette down with the liquid. It is important to avoid disturbing the buffy coat and the cell layers in the plasma tubes and clots in the serum tubes. If allowed, leave the bottom ~ 10% of plasma/serum behind to prevent cross-layer contamination.
If plasma/serum samples are to be aliquoted into more than one tube, it is important to transfer them from the blood collection tubes to a second, intermediary tube (such as low protein binding conical tubes) after centrifugation. Before aliquoting, the samples should be mixed by inverting the conical intermediary tube or pipetting up and down multiple times to ensure homogeneity. Direct aliquoting from the blood collection tubes right after centrifugation may lead to heterogeneity among aliquots due to the impact of centrifugation forces.
Hemolysis significantly deteriorates sample quality and is the primary cause of unusable specimens for clinical assays [ 140 ]. Therefore, samples should be inspected for signs of hemolysis which may impact the assay results. We recommend using a quick reference chart (such as the CDC Hemolysis Reference Palette) to record the hemolysis scale during specimen collection and checking the influence of hemolysis during data analysis.
Item 6: general procedures for serum collection
Figure 3 A illustrates the general procedures for collecting serum. We recommend collecting at least 5 ml of blood, yielding approximately 2.5 ml of serum to ensure sufficient specimens for multianalyte measurement. To minimize the impact of freeze/thaw, samples should be aliquoted. Both plain red-top tubes and serum separator tubes are commonly used for serum collection. Other than glass plain red top tubes, all other tube types require five inversions immediately after the blood draw to mix the blood with clot activators. Below are the general procedures for serum collection.
CRITICAL: If not using the glass red top tubes, phlebotomists should gently invert the blood tubes 5 times immediately after blood draw.
CRITICAL: Place the filled blood collection tubes upright at room temperature for 30 to 60 min to allow the clot to form.
CRITICAL: If the blood is not centrifuged immediately after the clotting time (30 to 60 min at room temperature), the tubes should be refrigerated (4 °C) for no longer than 2 h.
Centrifuge clotted tubes balanced by weight for 10 min at 1500 to 2000 × g at 4 °C.
Use the disposable transfer pipette to transfer the serum (top layer) to a 15 mL conical tube (or 50 mL conical tube if collecting 30 to 100 mL of blood). Be careful not to disturb the clot containing red blood cells, white blood cells, platelets, etc.
If more than one tube is collected, combine the serum samples from all tubes into the same conical tube.
Gently invert the conical tube 8–10 times to mix. Aliquot 250 μl to 1 ml into labeled microtubes or cryovials with O-ring-sealed screw leads. Residual aliquots can be saved and pooled as QC samples for repeated analysis.
Store all aliquots upright in a specimen box in an -80 °C or colder freezer.
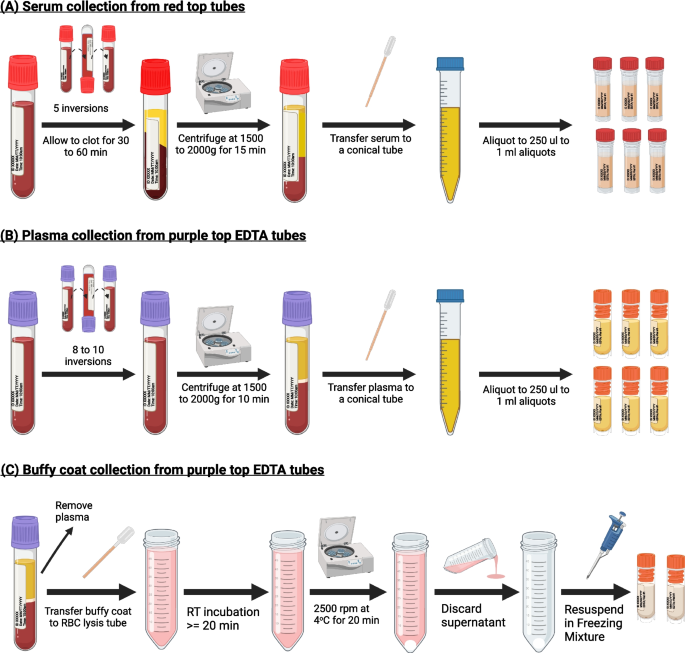
General procedures for serum ( A ), plasma ( B ), and buffy coat ( C ) collection from whole blood
Item 7: general procedures for plasma collection
Figure 3 B depicts the general procedures for collecting plasma. We recommend using EDTA or heparin tubes for blood collection, filling the tubes until the vacuum is exhausted, and following the procedures below.
CRITICAL: Immediately after blood collection, gently invert/mix (180-degree turns) the EDTA tubes 8–10 times. Place the tubes upright on a rack until centrifugation.
CRITICAL: It is advisable to store collected blood at 4 °C instead of at room temperature before centrifugation. Blood samples should be centrifuged within 2 h of blood collection to minimize degradation of AD biomarkers.
CRITICAL: In case of unavoidable prolonged centrifugation delay, place blood samples in the refrigerator for no more than 24 h. Avoid direct contact of blood tubes with ice to minimize cell lysis.
Centrifuge balanced blood collection tubes for 10 min at 1500 to 2000 × g at 4 °C.
Use the disposable transfer pipette to transfer the plasma (top layer) to a 15 mL conical tube (or 50 mL conical tube if collecting 30 to 100 mL of blood). Be careful not to disturb the buffy coat layer (the whitish layer in the middle) and the red blood cell layer (the red layer at the bottom).
If more than one tube is collected, combine the plasma samples from all blood collection tubes into the same conical tube.
Item 8: general procedures for buffy coat collection
Buffy coat contains most of the white blood cells and platelets of the anti-coagulated blood and is useful for a variety of clinical applications including genomic/genetic analysis. The remaining blood fractions after plasma collection can be used to collect buffy coat. To ensure high quality DNA, we recommend further enriching the buffy coats using a hypotonic solution to lyse the residual red blood cells. Figure 3 C illustrates the general procedures for buffy coat collection.
Note: Prepare the following reagents ahead of time and store them at 4 °C.
Ammonium chloride solution: 7.72 g/L
Ammonium bicarbonate solution: 0.79 g/L
Freezing mixture: TriPotassium Citrate: 17.8 g, Sodium Phosphate, monobasic: 2.4 g, Sodium Phosphate, dibasic: 2.8 g, Glycerin (Glycerol): 400 ml; bring volume to 1 L with distilled water.
Freshly prepare RBC lysis buffer by combining 45 ml ammonium chloride solution and 5 ml ammonium bicarbonate solution.
After removing the plasma (top layer) from the EDTA or heparin tubes, use another transfer pipette to draw the buffy coat (the whitish layer on top of the RBC layer) and place into the RBC lysis buffer tube (50 ml).
Mix by pipetting up and down to separate any leftover cells from within transfer pipette.
Cap the 50 ml tubes with lysis buffer + buffy coat and gently invert several times to mix.
Incubate at room temp for at least 20 min.
Add 10% bleach or Cavicide to the used blood tubes (lower layer with RBC) with leftover blood in them; discard in an appropriate biohazard bag.
After 20 min incubation, centrifuge 50 ml tubes at 4 °C for 20 min at 2500 rpm
After centrifuging, a white pellet will be visible at the bottom of the tube.
If no pellet is visible, centrifuge for an additional 20 min.
If pellet is visible, pour the red supernatant into a beaker filled with 10% bleach or Cavicide.
Let pellet dry (approximately 10–20 min).
Add 1 ml of freezing mixture to pellet. [ Freezing Mixture: TriPotassium Citrate: 17.8 g, Sodium Phosphate, monobasic: 2.4 g, Sodium Phosphate, dibasic: 2.8 g, Glycerin (Glycerol): 400 ml; bring volume to 1 L with distilled water].
Gently mix to break the pellet into single cell suspension.
Transfer whole (cells + freezing mix) into cryotubes.
Store at -80 °C for subsequent DNA isolation for genetic studies.
Item 9: detailed step-by-step SOP for blood collection and processing
We have combined the points discussed above and our own experiences to develop an SOP for the handling of blood for biomarker measurements (see Additional file 1 ). This SOP, which was primarily developed to streamline handling procedures for neurodegenerative disease cohort studies at the University of Pittsburgh Alzheimer’s Disease Research Center and ancillary centers and studies, can also be used by other investigators including those who are new to blood biomarker studies. Importantly, the SOP is adapted to allow for the concurrent processing of whole blood into plasma/serum for protein biomarker evaluation, and buffy coat for genetic/genomic studies. Moreover, the design of the SOP enables multiple tube aliquots to be collected and stored, and residual volumes pooled for quality control (QC) purposes.
Considerations for biobanking
Item 1: storage tubes and temperature.
To minimize analyte loss caused by adherence to the tube surfaces, it is recommended to use low protein binding microcentrifuge tubes or cryovials to store serum or plasma samples. For long-term storage, tubes with O-ring-sealed screws should be used to prevent evaporation. To prevent degradation, samples should be stored at ultra-low temperature freezers (-80 °C) or liquid N 2 tanks instead of -20 °C freezers. If feasible, aliquots of the same sample should be stored in separate freezers to prevent complete loss in the case of a freezer failure.
Item 2: freeze/thaw cycles
Several studies have investigated the impact of freeze/thaw cycles on blood biomarkers [ 107 , 116 , 141 , 142 , 143 ]. The overall results suggest that plasma AD biomarkers are stable for at least three freeze/thaw cycles. However, serum AD biomarkers may be more sensitive to freeze/thaw cycles. For example, serum Aβ40 level significantly decreased after any freeze/thaw cycle, while serum Aβ42 level showed a significant decrease at the third freeze-thaw cycle [ 107 ]. Therefore, to minimize the impact of freeze/thaw cycles, we recommend aliquoting samples in adequate volume, limiting the total number of freeze/thaw cycles to as few as possible, but never more than three.
Item 3: transportation
To transport samples to different facilities, it is recommended to use abundant dry ice sufficient to last at least 24 h post the expected delivery time to avoid sample thawing due to delivery delays. If possible, use a courier that replenishes dry ice mid-way, and avoid weekend delivery. It is worth noting that dry ice inside storage tubes may change the pH of the specimen during thawing and potentially influence the assays [ 144 ]. Therefore, samples may be stored free of dry ice in -80 °C freezers for at least 24 h before thawing for measurement. To provide guidance to readers, we have compiled a list of recommended practices during blood transportation, created based on issues regularly encountered at a major biomarker laboratory.
Include an accurate sample list (including accurate volumes). Ideally, provide a Microsoft Excel sheet (or similar) with columns for sample IDs, their corresponding volumes, and their location in the box. Adding a pictorial illustration of sample arrangements in the boxes is also helpful.
Inform the receiving laboratory ahead of time so that they will keep a lookout and be able to receive and store them in good time. The receiving lab may need to find freezer space before your samples arrive. Therefore, it is important that they are informed ahead of time.
Ensure that the package contains an adequate amount of dry ice. For long-distance transportation that spans multiple days, choose a reliable company that can refill the dry ice midway.
Print labels using a computer, rather than handwriting them, to ensure better legibility.
Use tubes with caps that do not become loose accidentally.
Sort the samples in the order you want them analyzed. If you are unsure of the order, there are two main rules:
If your samples are in groups: randomize them, so that all groups are represented in all analytical runs.
If you have longitudinal samples, keep all samples from the same participant together and in the order in which they were collected. Ensure your sample coding reflects this ordering.
Do the final sorting of the samples BEFORE you send them. It might take longer and be too time-consuming for the receiving lab to do it.
Considerations during biomarker measurements
The quality of laboratory and clinical assays has greatly improved due to advances in instrument technology, particularly the use of automated equipment, which has led to higher reproducibility through standardized procedures. However, analytical errors/variations from various sources may still occur and render results unusable or confound study findings. Common sources of analytical errors/variations include instrument malfunction, operators’ failure to follow procedures, inherent batch-to-batch variation of the assay, and matrix interference. To minimize errors/variations, it is important to use good process controls and follow good laboratory practices. Below, we outline several considerations that are critical for ensuring high-quality results and monitoring/maintaining the long-term stability of the AD blood biomarker measurements.
Item 1: preparation of samples for measurement
All blood specimens should be considered potential biohazards and handled with appropriate PPE. To ensure accurate measurement, it is crucial to homogenize samples before measurement. Samples should be completely thawed and mixed thoroughly. To minimize the impact of particulates that could clot cartridges or sample probes in the instrumentation, samples should be centrifuged before dispensing into a measurement container. It is also important to minimize the bubbles during pipetting. Any remaining samples should be promptly returned to the -80 °C freezer to minimize protein degradation. Place a dot on the tube lid with a permanent marker after each freeze-thaw cycle and keep track of the total number of cycles in updated inventory of samples. It is also recommended to keep samples with the same number of freeze-thaw cycles together and separate from the original, un-thawed samples.
Item 2: inclusion of calibrators
Despite significant technological advances, batch-to-batch variation remains an inherent issue in clinical chemistry. Contributing factors may include variations in instrument performance, changes in reagents and consumables, operator variability, etc. To address this issue, calibrators should be included in every batch to help correct for batch effects. Some platforms, such as Lumipulse, have been built to circumvent this issue such that there is an internal, manufacturer-provided calibration curve against which all sample results are plotted. However, this approach only works if the manufacturer can adjust all reagent batches to perform equivalently to the initial batch used to generate the built-in curve. The acceptance criteria for the calibration curve must be predefined to ensure accuracy and consistency. Although these criteria may vary depending on the platform used, certain parameters such as the regression coefficient, the variance between predicted and actual concentrations, and the repeatability for each calibrator should be considered.
Item 3: inclusion of QC samples
QC samples play a critical role in process control and should be included in every analytical run to evaluate assay performance and address potential errors. At a minimum, QC samples should be run in duplicates at the beginning and end of each analytical run. These samples should be selected in a way that they cover a range of concentrations across the standard curve or the typical range of concentrations for the measured sample values. It is typically advisable to use use three QC samples – low, average, and high concentrations relative to the assay standard curve. For optimal results, the same lot of QC samples should be used over time to help detect system or operator performance changes. Whenever possible, QC samples should be the same specimen types as the test samples. When switching between batches of QC samples, bridging is recommended – the relative comparison of QC samples to adjust the biomarker distribution on one plate to the other. These factors can then be applied to all samples measured on each plate/run to normalize the entire dataset. In Fig. 4 , we present a hypothetical example of a cohort consisting of 280 samples that were analyzed across four plates for Biomarker X. As shown in Fig. 4 A, the mean and overall data distribution of the uncorrected results from plates 1 and 2 appear similar, while plates 3 and 4 show a noticeable shift (simulation datasets in Additional file 2 ). However, after normalization using the plate-specific scaling factors based on QC readings, as shown in Fig. 4 B, the results from all four plates become more comparable.
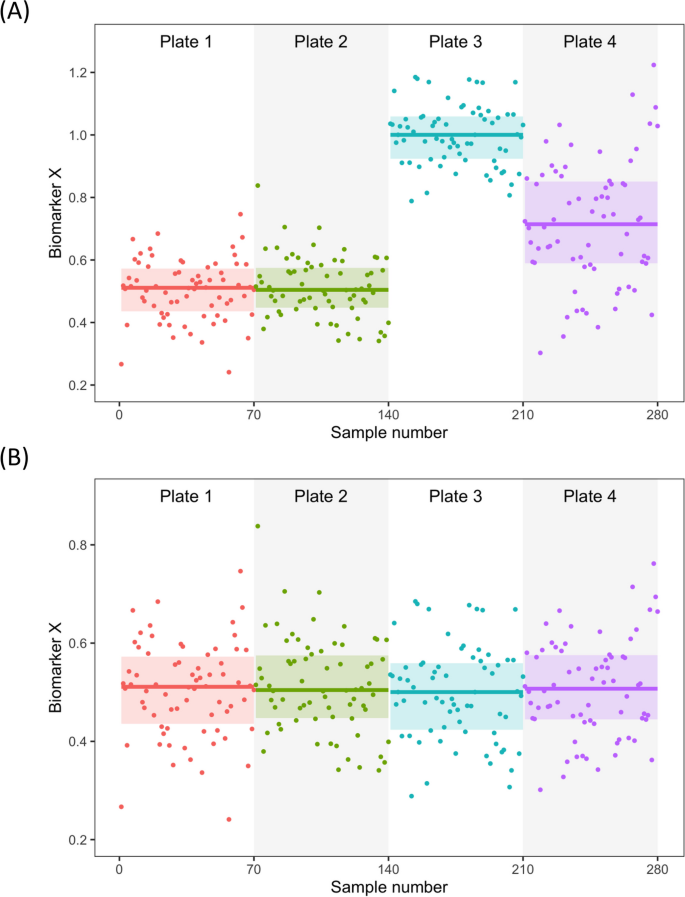
Visualization of data generated from measuring a hypothetical biomarker on 280 samples spread across four plates. The figure shows the results before ( A ) and after ( B ) adjustment based on the signals generated for identical aliquots of the same quality control samples measured on each plate, assuming all other key variables remain the same
Similar to calibrators, a predefined set of criteria should be applied to determine whether a run passes or fails. Key parameters to consider include the deviation from target values established through prior repeated analyses and the reproducibility of the QC measurements. An article by Schindler et al. [ 145 ] which tracked CSF assay performances over approximately a decade sheds light on this issue and potential corrective factors.
Item 4: sample measurement order
Batch-to-batch variation and within-batch run order effects can bias results, leading to erroneous conclusions. One way to address these issues is to randomize the order of sample analysis. There are various methods of randomization, including simple randomization, block randomization, stratified randomization, and covariate adaptive randomization [ 146 ]. Simple randomization involves using a single random sequence and is easy to implement, for example, by using the random number generator in Excel. However, simple randomization may result in an unequal distribution of groups and may not be suitable for studies with a small sample size ( n < 200). Block randomization addresses this issue by randomly assigning an equal number of samples from each group to each block and is more appropriate for small-size studies. For studies with multiple covariates, stratified randomization or covariate adaptive randomization may be necessary to control the potential uneven distribution of covariates. Additionally, for longitudinal measures, we recommend measuring all time points for the same participant simultaneously to minimize the impact of batch variation.
Item 5: sample blinding
Knowledge of grouping information may bias the analysis results and taint the data. Whenever possible, scientists performing the measurements and the analysts/statisticians who work with the ensuing data should be blinded from grouping allocation until measurement results have been finalized. A third party should perform the randomization to generate sample analysis orders.
Item 6: assay order
If multiple biomarkers are to be measured from the same aliquots, it is best practice to start with the biomarker most sensitive to degradation/denaturation from repeated freeze/thaw cycles. For example, among the core AD blood biomarkers, Aβ40 and Aβ42 tend to be most prone to degradation and should therefore be measured first, if possible, followed by t-tau, p-tau, BD-tau and finally GFAP and NfL. In addition, using multiplexed assays, if available, can help minimize the number of freeze/thaw cycles required.
Item 7: the use of bridging samples
For discovery research, it is recommended to process all samples at the same time with the same batch of reagents. However, in cases where it is unavoidable to change the reagent lots or run the analysis at different times or in various laboratories, harmonization can be achieved by repeating the measurement for bridging samples. Ideally, bridging samples should be from the same sample cohort and cover the full range of values of the study samples. The bridging samples are analyzed using the same reagents and consumables for each batch, allowing the determination of batch-specific normalization factors. The same approach described in Fig. 4 for normalizing run data from different plates is applicable here to between-batch normalization.
Item 8: longitudinal samples
Longitudinal studies, which involve continuous tracking of the same individuals over time, are commonly employed to assess the influence of various interventions or risk factors on disease outcomes. In contrast to cross-sectional studies, which capture snapshots of participants at a single point in time, longitudinal studies typically require an extended duration to collect specimens for the entire study. In some cases, researchers may be able to wait until all samples are collected to run them simultaneously. However, there are instances where running batches of samples along the way becomes necessary to gain preliminary insights into the data. To minimize the impact of temporal variation, proper control measures must be implemented to monitor and maintain the long-term stability of measurements. Several practical guidelines, proposed by Palmqvist et al. for AD CSF biomarkers, can be adopted for blood AD biomarkers [ 78 ]. These include controlling preanalytical variables, following good laboratory practices, utilizing calibrators and QC samples, and assessing the batch effect through batch-bridging. Ideally, one would like to use the same QCs throughout a longitudinal study. However, if this is not possible, it is important to perform batch-bridging and re-run samples from the previous analysis round to normalize runs. Therefore, it is crucial to properly plan for the longitudinal studies, including stocking up reagents (including QC and bridging samples) and consumables for the entire study if shelf life is allowed. While most blood AD biomarker research has been conducted in research settings, there is an increasing number of studies being initiated to evaluate the use of these biomarkers in real clinical practice, where specimens are continuously analyzed. Maintaining measurement stability becomes even more important in these real-world settings.
Considerations for result reporting
Item 1: demographic and clinical information.
To minimize potential bias caused by demographic and clinical factors, it is important to include the distribution of available demographic and clinical characteristics among groups in the report, along with their associated statistical findings. These information help identify potential moderating variables and adjust for their effect in the analysis. Additionally, including this information in the report allows better comparison with results from different studies.
Item 2: full description of methods
To facilitate the coherent pooling of data in future meta-analyses, it is important to include detailed descriptions of blood collection/handling protocols and laboratory assay protocols in the Method section of peer-reviewed publications. In addition, as preanalytical variables, such as blood draw devices and parameters during blood processing, may interfere with AD biomarker measurements, it is important to describe these variables and to indicated if they were changed during the study.
Item 3: disclosure of assay performance
It is important to include assay performance in the publication to enable other researchers to evaluate the quality of the reported results. Multiple attributes can be useful for assessing assay performance, such as calibration curves’ R -value, linearity range, the limit of detection, and limit of quantification, as well as QC performance measures such as repeatability and intermediate precision. Moreover, the reports should also disclose if plate adjustments, such as with the use of bridging samples or QC samples, have been performed to account for batch variation.
Unresolved issues
Certified reference materials.
A major source of confusion in the field is that blood biomarker assays for the same analyte (e.g., plasma p-tau181 or p-tau217) from various sources return very different numerical values in terms of concentrations. We have sought to explain the analytical reasons behind this phenomenon – that it is often due to the use of different calibrators as well as variability of signals from the technical platforms [ 13 ]. However, the issue limits the comparability of assay results across platforms, which might become even more acute once cutpoints are generated. The use of certified reference materials could provide a solution, by providing a focal point for comparing and calibrating the standards used in the different assays. An example of the use of certified reference materials in mitigating between-assay bias was demonstrated by the application by Boulo S et al. in CSF samples [ 147 ].
Real world applications
Despite excellent performances of blood biomarkers demonstrated in many publications, we should be mindful that the results have mostly come from highly selected research cohorts that additionally have prior confirmation of pathology using CSF or neuroimaging biomarkers or even sometimes both. As we venture into applying blood biomarkers as potential first line screeners in clinical practice and therapeutic trials, it is important to note that this might be the real “test drive” for the biomarkers. As such, their performances may not be as stellar as observed for multiple reasons. This includes the much lower prevalence of AD pathophysiology in the community compared with the research cohorts, and the high heterogeneity of diseases presented in the clinics contrary to those included in defined cohorts. Moreover, such heterogenous cases would not benefit from prior categorization using CSF and neuroimaging biomarkers as often done for the existing cohorts that have recorded high accuracies.
Clinical use of blood biomarkers is much anticipated. However, it is often forgotten that one of the fundamental factors that need addressing prior to this happening is the generation and validation of assay thresholds. Such threshold points would not only be based on the detection of amyloid and/or tau pathology but would additionally investigate potential clinical scenarios such as if and how the set cutpoints respond to given demographic and clinical variables.
The blood AD biomarker research field has experienced rapid growth in recent years, with numerous studies highlighting the potential of blood-based AD biomarkers in supporting clinical decision-making and accelerating therapeutic development. We have outlined in this review general guidelines for the various steps involved in blood AD biomarker measurement, to promote good laboratory practices to minimize analytical errors and facilitate the development of standardized protocols that can improve reproducibility and enable cross-validation across different research centers. Table 2 provides a summary of the important preanalytical, analytical and post-analytical factors to consider in AD blood biomarker research. However, further research is needed to fully understand the impact of preanalytical and analytical variables, and these guidelines should be updated as new research findings become available. Finally, we provide an adaptable SOP that can be applied to blood collection, processing, and downstream handling in biomarker evaluations.
Availability of data and materials
All the data are included in the manuscript and the supporting information.
Abbreviations
Amyloid-beta
- Alzheimer’s disease
Brain-derived tau
Cerebrospinal fluid
Dried blood spot
Dried plasma spot
Electrochemiluminescence
Ethylenediaminetetraacetic acid
Enzyme-linked immunosorbent assay
General Data Protection Regulation
Glial fibrillary acidic protein
Good laboratory practice
Health Insurance Portability and Accountability Act of 1996
Interdigitated microelectrode sensor
Immunomagnetic reduction
Immunoprecipitation coupled with mass spectrometry
Intravenous
Magnetic resonance imaging
Mass spectrometry
Meso Scale Discovery
Neurofilament light-chain
Phosphorylated tau
Positron emission tomography
Personal protective equipment
Quality control
Single-molecule array
Standardized operating procedure
Serum separator tubes
Alzheimer’s Association. 2023. Alzheimer’s disease facts and figures. Alzheimers Dement. 2023;19:1598–695.
Hebert LE, Weuve J, Scherr PA, Evans DA. Alzheimer disease in the United States (2010–2050) estimated using the 2010 census. Neurology. 2013;80:1778–83.
Article PubMed PubMed Central Google Scholar
Patterson C. World Alzheimer Report 2018: The state of the art of dementia research; New frontiers. London: Alzheimer’s Disease International; 2018.
Alzheimer A, Stelzmann RA, Schnitzlein HN, Murtagh FR. An English translation of Alzheimer’s 1907 paper, “Uber eine eigenartige Erkankung der Hirnrinde.” Clin Anat. 1995;8:429–31.
Article CAS PubMed Google Scholar
DeTure MA, Dickson DW. The neuropathological diagnosis of Alzheimer’s disease. Mol Neurodegener. 2019;14:32.
Tarawneh R, Holtzman DM. The clinical problem of symptomatic Alzheimer disease and mild cognitive impairment. Cold Spring Harb Perspect Med. 2012;2:a006148.
van Dyck CH, Swanson CJ, Aisen P, Bateman RJ, Chen C, Gee M, Kanekiyo M, Li D, Reyderman L, Cohen S, et al. Lecanemab in early Alzheimer’s disease. N Engl J Med. 2022;388:9–21.
Article PubMed Google Scholar
Budd Haeberlein S, Aisen PS, Barkhof F, Chalkias S, Chen T, Cohen S, Dent G, Hansson O, Harrison K, von Hehn C, et al. Two randomized phase 3 studies of aducanumab in early Alzheimer’s disease. J Prev Alzheimers Dis. 2022;9:197–210.
CAS PubMed Google Scholar
Reardon S. FDA approves Alzheimer’s drug lecanemab amid safety concerns. Nature. 2023;613:227–8.
Jack CR Jr, Bennett DA, Blennow K, Carrillo MC, Dunn B, Haeberlein SB, Holtzman DM, Jagust W, Jessen F, Karlawish J, et al. NIA-AA research framework: toward a biological definition of Alzheimer’s disease. Alzheimers Dement. 2018;14:535–62.
Alawode DOT, Heslegrave AJ, Ashton NJ, Karikari TK, Simrén J, Montoliu-Gaya L, Pannee J, O’Connor A, Weston PSJ, Lantero-Rodriguez J, et al. Transitioning from cerebrospinal fluid to blood tests to facilitate diagnosis and disease monitoring in Alzheimer’s disease. J Intern Med. 2021;290:583–601.
Article CAS PubMed PubMed Central Google Scholar
Balogun WG, Zetterberg H, Blennow K, Karikari TK. Plasma biomarkers for neurodegenerative disorders: ready for prime time? Curr Opin Psychiatry. 2023;36(2):112.
Karikari TK, Ashton NJ, Brinkmalm G, Brum WS, Benedet AL, Montoliu-Gaya L, Lantero-Rodriguez J, Pascoal TA, Suárez-Calvet M, Rosa-Neto P, et al. Blood phospho-tau in Alzheimer disease: analysis, interpretation, and clinical utility. Nat Rev Neurol. 2022;18:400–18.
Malzbender K, Lavin-Mena L, Hughes L, Bose N, Goldman D, Patel D. Key Barriers to Clinical Trials for Alzheimer’s Disease. Leonard D., editor. Los Angeles, CA, USA: Schaeffer Center for Health Policy and Economics; 2020.
Moscoso A, Karikari TK, Grothe MJ, Ashton NJ, Lantero-Rodriguez J, Snellman A, Zetterberg H, Blennow K, Schöll M. CSF biomarkers and plasma p-tau181 as predictors of longitudinal tau accumulation: Implications for clinical trial design. Alzheimers Dement. 2022;18:2614–26.
Cullen NC, Leuzy A, Janelidze S, Palmqvist S, Svenningsson AL, Stomrud E, Dage JL, Mattsson-Carlgren N, Hansson O. Plasma biomarkers of Alzheimer’s disease improve prediction of cognitive decline in cognitively unimpaired elderly populations. Nat Commun. 2021;12:3555.
Rissin DM, Kan CW, Campbell TG, Howes SC, Fournier DR, Song L, Piech T, Patel PP, Chang L, Rivnak AJ, et al. Single-molecule enzyme-linked immunosorbent assay detects serum proteins at subfemtomolar concentrations. Nat Biotechnol. 2010;28:595–9.
Nakamura A, Kaneko N, Villemagne VL, Kato T, Doecke J, Doré V, Fowler C, Li Q-X, Martins R, Rowe C, et al. High performance plasma amyloid-β biomarkers for Alzheimer’s disease. Nature. 2018;554:249–54.
Ovod V, Ramsey KN, Mawuenyega KG, Bollinger JG, Hicks T, Schneider T, Sullivan M, Paumier K, Holtzman DM, Morris JC, et al. Amyloid β concentrations and stable isotope labeling kinetics of human plasma specific to central nervous system amyloidosis. Alzheimers Dement. 2017;13:841–9.
Chong JR, Ashton NJ, Karikari TK, Tanaka T, Schöll M, Zetterberg H, Blennow K, Chen CP, Lai MKP. Blood-based high sensitivity measurements of beta-amyloid and phosphorylated tau as biomarkers of Alzheimer’s disease: a focused review on recent advances. J Neurol Neurosurg Psychiatry. 2021;92:1231–41.
Gonzalez-Ortiz F, Turton M, Kac PR, Smirnov D, Premi E, Ghidoni R, Benussi L, Cantoni V, Saraceno C, Rivolta J, et al. Brain-derived tau: a novel blood-based biomarker for Alzheimer’s disease-type neurodegeneration. Brain. 2023;146:1152–65.
Pereira JB, Janelidze S, Smith R, Mattsson-Carlgren N, Palmqvist S, Zetterberg H, Stomrud E, Ashton NJ, Blennow K, Hansson O. Plasma glial fibrillary acidic protein is an early marker of Aβ pathology in Alzheimer’s disease. medRxiv. 2021:2021.2004.2011.21255152.
Osborn LM, Kamphuis W, Wadman WJ, Hol EM. Astrogliosis: an integral player in the pathogenesis of Alzheimer’s disease. Prog Neurobiol. 2016;144:121–41.
Bellaver B, Povala G, Ferreira PCL, Ferrari-Souza JP, Leffa DT, Lussier FZ, Benedet AL, Ashton NJ, Triana-Baltzer G, Kolb HC, et al. Astrocyte reactivity influences amyloid-β effects on tau pathology in preclinical Alzheimer’s disease. Nat Med. 2023;29(7):1775–81.
Benedet AL, Brum WS, Hansson O, Karikari TK, Zimmer ER, Zetterberg H, Blennow K, Ashton NJ. The accuracy and robustness of plasma biomarker models for amyloid PET positivity. Alzheimers Res Ther. 2022;14:26.
Verberk IMW, Misdorp EO, Koelewijn J, Ball AJ, Blennow K, Dage JL, Fandos N, Hansson O, Hirtz C, Janelidze S, et al. Characterization of pre-analytical sample handling effects on a panel of Alzheimer’s disease–related blood-based biomarkers: Results from the Standardization of Alzheimer’s Blood Biomarkers (SABB) working group. Alzheimers Dement. 2022;18:1484–97.
Plebani M. Exploring the iceberg of errors in laboratory medicine. Clin Chim Acta. 2009;404:16–23.
Teunissen CE, Petzold A, Bennett JL, Berven FS, Brundin L, Comabella M, Franciotta D, Frederiksen JL, Fleming JO, Furlan R, et al. A consensus protocol for the standardization of cerebrospinal fluid collection and biobanking. Neurology. 2009;73:1914–22.
Gobom J, Parnetti L, Rosa-Neto P, Vyhnalek M, Gauthier S, Cataldi S, Lerch O, Laczo J, Cechova K, Clarin M, et al. Validation of the LUMIPULSE automated immunoassay for the measurement of core AD biomarkers in cerebrospinal fluid. Clin Chem Lab Med. 2022;60:207–19.
Schindler SE, Bollinger JG, Ovod V, Mawuenyega KG, Li Y, Gordon BA, Holtzman DM, Morris JC, Benzinger TLS, Xiong C, et al. High-precision plasma β-amyloid 42/40 predicts current and future brain amyloidosis. Neurology. 2019;93:e1647–59.
O’Bryant SE, Gupta V, Henriksen K, Edwards M, Jeromin A, Lista S, Bazenet C, Soares H, Lovestone S, Hampel H, et al. Guidelines for the standardization of preanalytic variables for blood-based biomarker studies in Alzheimer’s disease research. Alzheimers Dement. 2015;11:549–60.
Armstrong RA. Risk factors for Alzheimer’s disease. Folia Neuropathol. 2019;57:87–105.
Article Google Scholar
Henderson AS. The risk factors for Alzheimer’s disease: a review and a hypothesis. Acta Psychiatr Scand. 1988;78:257–75.
Killin LOJ, Starr JM, Shiue IJ, Russ TC. Environmental risk factors for dementia: a systematic review. BMC Geriatr. 2016;16:175.
Mielke MM, Dage JL, Frank RD, Algeciras-Schimnich A, Knopman DS, Lowe VJ, Bu G, Vemuri P, Graff-Radford J, Jack CR Jr, Petersen RC. Performance of plasma phosphorylated tau 181 and 217 in the community. Nat Med. 2022;28:1398–405.
Toledo JB, Vanderstichele H, Figurski M, Aisen PS, Petersen RC, Weiner MW, Jack CR, Jagust W, Decarli C, Toga AW, et al. Factors affecting Aβ plasma levels and their utility as biomarkers in ADNI. Acta Neuropathol. 2011;122:401.
Schindler SE, Karikari TK, Ashton NJ, Henson RL, Yarasheski KE, West T, Meyer MR, Kirmess KM, Li Y, Saef B, et al. Effect of race on prediction of brain amyloidosis by plasma Aβ42/Aβ40, phosphorylated tau, and neurofilament light. Neurology. 2022;99:e245–57.
Syrjanen JA, Campbell MR, Algeciras-Schimnich A, Vemuri P, Graff-Radford J, Machulda MM, Bu G, Knopman DS, Jack CR Jr, Petersen RC, Mielke MM. Associations of amyloid and neurodegeneration plasma biomarkers with comorbidities. Alzheimers Dement. 2022;18:1128–40.
Arvanitakis Z, Lucas JA, Younkin LH, Younkin SG, Graff-Radford NR. Serum creatinine levels correlate with plasma amyloid β protein. Alzheimer Dis Assoc Disord. 2002;16:187–90.
Blasko I, Kemmler G, Krampla W, Jungwirth S, Wichart I, Jellinger K, Tragl KH, Fischer P. Plasma amyloid beta protein 42 in non-demented persons aged 75 years: effects of concomitant medication and medial temporal lobe atrophy. Neurobiol Aging. 2005;26:1135–43.
Chatterjee P, Pedrini S, Ashton NJ, Tegg M, Goozee K, Singh AK, Karikari TK, Simrén J, Vanmechelen E, Armstrong NJ, et al. Diagnostic and prognostic plasma biomarkers for preclinical Alzheimer’s disease. Alzheimers Dement. 2022;18:1141–54.
Lopez OL, Chang Y, Ives DG, Snitz BE, Fitzpatrick AL, Carlson MC, Rapp SR, Williamson JD, Tracy RP, DeKosky ST, Kuller LH. Blood amyloid levels and risk of dementia in the Ginkgo Evaluation of Memory Study (GEMS): a longitudinal analysis. Alzheimers Dement. 2019;15:1029–38.
Pontecorvo MJ, Lu M, Burnham SC, Schade AE, Dage JL, Shcherbinin S, Collins EC, Sims JR, Mintun MA. Association of donanemab treatment with exploratory plasma biomarkers in early symptomatic Alzheimer disease: a secondary analysis of the TRAILBLAZER-ALZ randomized clinical trial. JAMA Neurol. 2022;79:1250–9.
Depypere H, Vergallo A, Lemercier P, Lista S, Benedet A, Ashton N, Cavedo E, Zetterberg H, Blennow K, Vanmechelen E, et al. Menopause hormone therapy significantly alters pathophysiological biomarkers of Alzheimer’s disease. Alzheimers Dement. 2023;19:1320–30.
Thurston RC, Maki P, Chang Y, Wu M, Aizenstein HJ, Derby CA, Karikari TK. Menopausal vasomotor symptoms and plasma Alzheimer disease biomarkers. Am J Obstet Gynecol. 2023. https://doi.org/10.1016/j.ajog.2023.11.002 .
Sakpal TV. Sample size estimation in clinical trial. Perspect Clin Res. 2010;1:67–9.
PubMed PubMed Central Google Scholar
Milà-Alomà M, Ashton NJ, Shekari M, Salvadó G, Ortiz-Romero P, Montoliu-Gaya L, Benedet AL, Karikari TK, Lantero-Rodriguez J, Vanmechelen E, et al. Plasma p-tau231 and p-tau217 as state markers of amyloid-β pathology in preclinical Alzheimer’s disease. Nat Med. 2022;28:1797–801.
Fandos N, Pérez-Grijalba V, Pesini P, Olmos S, Bossa M, Villemagne VL, Doecke J, Fowler C, Masters CL, Sarasa M. Plasma amyloid β 42/40 ratios as biomarkers for amyloid β cerebral deposition in cognitively normal individuals. Alzheimers Dement (Amst). 2017;8:179–87.
De Meyer S, Schaeverbeke JM, Verberk IMW, Gille B, De Schaepdryver M, Luckett ES, Gabel S, Bruffaerts R, Mauroo K, Thijssen EH, et al. Comparison of ELISA- and SIMOA-based quantification of plasma Aβ ratios for early detection of cerebral amyloidosis. Alzheimers Res Ther. 2020;12:162.
Doecke JD, Pérez-Grijalba V, Fandos N, Fowler C, Villemagne VL, Masters CL, Pesini P, Sarasa M. Total Aβ(42)/Aβ(40) ratio in plasma predicts amyloid-PET status, independent of clinical AD diagnosis. Neurology. 2020;94:e1580–91.
Vogelgsang J, Shahpasand-Kroner H, Vogelgsang R, Streit F, Vukovich R, Wiltfang J. Multiplex immunoassay measurement of amyloid-β42 to amyloid-β40 ratio in plasma discriminates between dementia due to Alzheimer’s disease and dementia not due to Alzheimer’s disease. Exp Brain Res. 2018;236:1241–50.
Palmqvist S, Janelidze S, Stomrud E, Zetterberg H, Karl J, Zink K, Bittner T, Mattsson N, Eichenlaub U, Blennow K, Hansson O. Performance of fully automated plasma assays as screening tests for Alzheimer disease-related β-amyloid status. JAMA Neurol. 2019;76:1060–9.
Wilson DH, Rissin DM, Kan CW, Fournier DR, Piech T, Campbell TG, Meyer RE, Fishburn MW, Cabrera C, Patel PP, et al. The Simoa HD-1 analyzer: a novel fully automated digital immunoassay analyzer with single-molecule sensitivity and multiplexing. J Lab Autom. 2016;21:533–47.
Keshavan A, Pannee J, Karikari TK, Rodriguez JL, Ashton NJ, Nicholas JM, Cash DM, Coath W, Lane CA, Parker TD, et al. Population-based blood screening for preclinical Alzheimer’s disease in a British birth cohort at age 70. Brain. 2021;144:434–49.
Aldo P, Marusov G, Svancara D, David J, Mor G. Simple Plex(™): a novel multi-analyte, automated microfluidic immunoassay platform for the detection of human and mouse cytokines and chemokines. Am J Reprod Immunol. 2016;75:678–93.
Kaneko N, Nakamura A, Washimi Y, Kato T, Sakurai T, Arahata Y, Bundo M, Takeda A, Niida S, Ito K, et al. Novel plasma biomarker surrogating cerebral amyloid deposition. Proc Jpn Acad Ser B Phys Biol Sci. 2014;90:353–64.
Kirmess KM, Meyer MR, Holubasch MS, Knapik SS, Hu Y, Jackson EN, Harpstrite SE, Verghese PB, West T, Fogelman I, et al. The PrecivityAD™ test: accurate and reliable LC-MS/MS assays for quantifying plasma amyloid beta 40 and 42 and apolipoprotein E proteotype for the assessment of brain amyloidosis. Clin Chim Acta. 2021;519:267–75.
Hong C-Y, Wu CC, Chiu YC, Yang SY, Horng HE, Yang HC. Magnetic susceptibility reduction method for magnetically labeled immunoassay. Appl Phys Lett. 2006;88:212512.
Chieh JJ, Yang SY, Jian ZF, Wang WC, Horng HE, Yang HC, Hong C-Y. Hyper-high-sensitivity wash-free magnetoreduction assay on biomolecules using high-Tc superconducting quantum interference devices. J Appl Phys. 2008;103:014703.
Yang Y-H, Situmeang RFV, Ong PA. Can blood amyloid levels be used as a biomarker for Alzheimer’s disease? Brain Sci Adv. 2021;7:17–25.
Lue L-F, Kuo Y-M, Sabbagh M. Advance in plasma AD core biomarker development: current findings from immunomagnetic reduction-based SQUID technology. Neurol Ther. 2019;8:95–111.
Kim Y, Yoo YK, Kim HY, Roh JH, Kim J, Baek S, Lee JC, Kim HJ, Chae M-S, Jeong D, et al. Comparative analyses of plasma amyloid-β levels in heterogeneous and monomerized states by interdigitated microelectrode sensor system. Sci Adv. 2019;5:eaav1388.
Wilson EN, Young CB, Ramos Benitez J, Swarovski MS, Feinstein I, Vandijck M, Le Guen Y, Kasireddy NM, Shahid M, Corso NK, et al. Performance of a fully-automated Lumipulse plasma phospho-tau181 assay for Alzheimer’s disease. Alzheimers Res Ther. 2022;14:172.
Gauthier A, Viel S, Perret M, Brocard G, Casey R, Lombard C, Laurent-Chabalier S, Debouverie M, Edan G, Vukusic S, et al. Comparison of SimoaTM and EllaTM to assess serum neurofilament-light chain in multiple sclerosis. Ann Clin Transl Neurol. 2021;8:1141–50.
Truffi M, Garofalo M, Ricciardi A, Cotta Ramusino M, Perini G, Scaranzin S, Gastaldi M, Albasini S, Costa A, Chiavetta V, et al. Neurofilament-light chain quantification by Simoa and Ella in plasma from patients with dementia: a comparative study. Sci Rep. 2023;13:4041.
Ding XL, Tuo QZ, Lei P. An introduction to ultrasensitive assays for plasma tau detection. J Alzheimers Dis. 2021;80:1353–62.
Lue LF, Guerra A, Walker DG. Amyloid beta and tau as Alzheimer’s disease blood biomarkers: promise from new technologies. Neurol Ther. 2017;6:25–36.
Li D, Mielke MM. An update on blood-based markers of Alzheimer’s disease using the SiMoA platform. Neurol Ther. 2019;8:73–82.
Barthélemy NR, Horie K, Sato C, Bateman RJ. Blood plasma phosphorylated-tau isoforms track CNS change in Alzheimer’s disease. J Exp Med. 2020;217(11):e20200861.
Jang H, Kim JS, Lee HJ, Kim C-H, Na DL, Kim HJ, Allué JA, Sarasa L, Castillo S, Pesini P, et al. Performance of the plasma Aβ42/Aβ40 ratio, measured with a novel HPLC-MS/MS method, as a biomarker of amyloid PET status in a DPUK-KOREAN cohort. Alzheimers Res Ther. 2021;13:179.
Montoliu-Gaya L, Benedet AL, Tissot C, Vrillon A, Ashton NJ, Brum WS, Lantero-Rodriguez J, Stevenson J, Nilsson J, Sauer M, et al. Mass spectrometric simultaneous quantification of tau species in plasma shows differential associations with amyloid and tau pathologies. Nat Aging. 2023;3:661–9.
Palmqvist S, Stomrud E, Cullen N, Janelidze S, Manuilova E, Jethwa A, Bittner T, Eichenlaub U, Suridjan I, Kollmorgen G, et al. An accurate fully automated panel of plasma biomarkers for Alzheimer’s disease. Alzheimers Dement. 2023;19:1204–15.
Feng W, Beer JC, Hao Q, Ariyapala IS, Sahajan A, Komarov A, Cha K, Moua M, Qiu X, Xu X, et al. NULISA: a proteomic liquid biopsy platform with attomolar sensitivity and high multiplexing. Nat Commun. 2023;14:7238.
Yang S-Y, Chiu M-J, Chen T-F, Horng H-E. Detection of plasma biomarkers using immunomagnetic reduction: a promising method for the early diagnosis of Alzheimer’s disease. Neurol Ther. 2017;6:37–56.
Pannee J, Shaw LM, Korecka M, Waligorska T, Teunissen CE, Stoops E, Vanderstichele HMJ, Mauroo K, Verberk IMW, Keshavan A, et al. The global Alzheimer’s Association round robin study on plasma amyloid β methods. Alzheimers Dement (Amst). 2021;13:e12242.
Korecka M, Shaw LM. Mass spectrometry-based methods for robust measurement of Alzheimer’s disease biomarkers in biological fluids. J Neurochem. 2021;159:211–33.
Palmqvist S, Zetterberg H, Mattsson N, Johansson P, Minthon L, Blennow K, Olsson M, Hansson O. Detailed comparison of amyloid PET and CSF biomarkers for identifying early Alzheimer disease. Neurology. 2015;85:1240–9.
Palmqvist S, Zetterberg H, Blennow K, Vestberg S, Andreasson U, Brooks DJ, Owenius R, Hägerström D, Wollmer P, Minthon L, Hansson O. Accuracy of brain amyloid detection in clinical practice using cerebrospinal fluid β-amyloid 42: a cross-validation study against amyloid positron emission tomography. JAMA Neurol. 2014;71:1282–9.
Qu Y, Ma Y-H, Huang Y-Y, Ou Y-N, Shen X-N, Chen S-D, Dong Q, Tan L, Yu J-T. Blood biomarkers for the diagnosis of amnestic mild cognitive impairment and Alzheimer’s disease: a systematic review and meta-analysis. Neurosci Biobehav Rev. 2021;128:479–86.
Koychev I, Jansen K, Dette A, Shi L, Holling H. Blood-based ATN biomarkers of Alzheimer’s disease: a meta-analysis. J Alzheimers Dis. 2021;79:177–95.
Janelidze S, Teunissen CE, Zetterberg H, Allué JA, Sarasa L, Eichenlaub U, Bittner T, Ovod V, Verberk IMW, Toba K, et al. Head-to-head comparison of 8 plasma amyloid-β 42/40 assays in Alzheimer disease. JAMA Neurol. 2021;78:1375–82.
Mattsson N, Insel PS, Palmqvist S, Portelius E, Zetterberg H, Weiner M, Blennow K, Hansson O. Initiative tAsDN: cerebrospinal fluid tau, neurogranin, and neurofilament light in Alzheimer’s disease. EMBO Mol Med. 2016;8:1184–96.
Zetterberg H, Wilson D, Andreasson U, Minthon L, Blennow K, Randall J, Hansson O. Plasma tau levels in Alzheimer’s disease. Alzheimers Res Ther. 2013;5:9.
Mattsson N, Zetterberg H, Janelidze S, Insel PS, Andreasson U, Stomrud E, Palmqvist S, Baker D, Tan Hehir CA, Jeromin A, et al. Plasma tau in Alzheimer disease. Neurology. 2016;87:1827–35.
Chen Z, Mengel D, Keshavan A, Rissman RA, Billinton A, Perkinton M, Percival-Alwyn J, Schultz A, Properzi M, Johnson K, et al. Learnings about the complexity of extracellular tau aid development of a blood-based screen for Alzheimer’s disease. Alzheimers Dement. 2019;15:487–96.
Snellman A, Lantero-Rodriguez J, Emeršič A, Vrillon A, Karikari TK, Ashton NJ, Gregorič Kramberger M, Čučnik S, Paquet C, Rot U, et al. N-terminal and mid-region tau fragments as fluid biomarkers in neurological diseases. Brain. 2022;145:2834–48.
Ashton NJ, Benedet AL, Pascoal TA, Karikari TK, Lantero-Rodriguez J, Brum WS, Mathotaarachchi S, Therriault J, Savard M, Chamoun M, et al. Cerebrospinal fluid p-tau231 as an early indicator of emerging pathology in Alzheimer’s disease. eBioMedicine. 2022;76:103836.
Karikari TK, Emeršič A, Vrillon A, Lantero-Rodriguez J, Ashton NJ, Kramberger MG, Dumurgier J, Hourregue C, Čučnik S, Brinkmalm G, et al. Head-to-head comparison of clinical performance of CSF phospho-tau T181 and T217 biomarkers for Alzheimer’s disease diagnosis. Alzheimers Dement. 2021;17:755–67.
Karikari TK, Pascoal TA, Ashton NJ, Janelidze S, Benedet AL, Rodriguez JL, Chamoun M, Savard M, Kang MS, Therriault J, et al. Blood phosphorylated tau 181 as a biomarker for Alzheimer’s disease: a diagnostic performance and prediction modelling study using data from four prospective cohorts. Lancet Neurol. 2020;19:422–33.
Karikari TK, Benedet AL, Ashton NJ, Lantero Rodriguez J, Snellman A, Suárez-Calvet M, Saha-Chaudhuri P, Lussier F, Kvartsberg H, Rial AM, et al. Diagnostic performance and prediction of clinical progression of plasma phospho-tau181 in the Alzheimer’s Disease Neuroimaging Initiative. Mol Psychiatry. 2021;26:429–42.
Ashton NJ, Pascoal TA, Karikari TK, Benedet AL, Lantero-Rodriguez J, Brinkmalm G, Snellman A, Schöll M, Troakes C, Hye A, et al. Plasma p-tau231: a new biomarker for incipient Alzheimer’s disease pathology. Acta Neuropathol. 2021;141:709–24.
Palmqvist S, Janelidze S, Quiroz YT, Zetterberg H, Lopera F, Stomrud E, Su Y, Chen Y, Serrano GE, Leuzy A, et al. Discriminative accuracy of plasma phospho-tau217 for Alzheimer disease vs other neurodegenerative disorders. JAMA. 2020;324:772–81.
Mielke MM, Frank RD, Dage JL, Jeromin A, Ashton NJ, Blennow K, Karikari TK, Vanmechelen E, Zetterberg H, Algeciras-Schimnich A, et al. Comparison of plasma phosphorylated tau species with amyloid and tau positron emission tomography, neurodegeneration, vascular pathology, and cognitive outcomes. JAMA Neurol. 2021;78:1108–17.
Ashton NJ, Puig-Pijoan A, Milà-Alomà M, Fernández-Lebrero A, García-Escobar G, González-Ortiz F, Kac PR, Brum WS, Benedet AL, Lantero-Rodriguez J, et al. Plasma and CSF biomarkers in a memory clinic: Head-to-head comparison of phosphorylated tau immunoassays. Alzheimers Dement. 2023;19:1913–24.
Bayoumy S, Verberk IMW, den Dulk B, Hussainali Z, Zwan M, van der Flier WM, Ashton NJ, Zetterberg H, Blennow K, Vanbrabant J, et al. Clinical and analytical comparison of six Simoa assays for plasma P-tau isoforms P-tau181, P-tau217, and P-tau231. Alzheimers Res Ther. 2021;13:198.
Janelidze S, Bali D, Ashton NJ, Barthélemy NR, Vanbrabant J, Stoops E, Vanmechelen E, He Y, Dolado AO, Triana-Baltzer G, et al. Head-to-head comparison of 10 plasma phospho-tau assays in prodromal Alzheimer’s disease. Brain. 2023;146:1592–601.
Kac PR, González-Ortiz F, Emeršič A, Dulewicz M, Koutarapu S, Turton M, An Y, Smirnov D, Kulczyńska-Przybik A, Varma V, et al. Plasma p-tau212: antemortem diagnostic performance and prediction of autopsy verification of Alzheimer’s disease neuropathology. medRxiv. 2023:2023.2012.2011.23299806.
Ashton NJ, Janelidze S, Mattsson-Carlgren N, Binette AP, Strandberg O, Brum WS, Karikari TK, González-Ortiz F, Di Molfetta G, Meda FJ, et al. Differential roles of Aβ42/40, p-tau231 and p-tau217 for Alzheimer’s trial selection and disease monitoring. Nat Med. 2022;28:2555–62.
Yang Z, Wang KK. Glial fibrillary acidic protein: from intermediate filament assembly and gliosis to neurobiomarker. Trends Neurosci. 2015;38:364–74.
Monterey MD, Wei H, Wu X, Wu JQ. The many faces of astrocytes in Alzheimer’s disease. Front Neurol. 2021;12:619626.
Chatterjee P, Pedrini S, Stoops E, Goozee K, Villemagne VL, Asih PR, Verberk IMW, Dave P, Taddei K, Sohrabi HR, et al. Plasma glial fibrillary acidic protein is elevated in cognitively normal older adults at risk of Alzheimer’s disease. Transl Psychiatry. 2021;11:27.
Benedet AL, Milà-Alomà M, Vrillon A, Ashton NJ, Pascoal TA, Lussier F, Karikari TK, Hourregue C, Cognat E, Dumurgier J, et al. Differences between plasma and cerebrospinal fluid glial fibrillary acidic protein levels across the Alzheimer disease continuum. JAMA Neurol. 2021;78:1471–83.
Papa L, Ladde JG, O’Brien JF, Thundiyil JG, Tesar J, Leech S, Cassidy DD, Roa J, Hunter C, Miller S, et al. Evaluation of glial and neuronal blood biomarkers compared with clinical decision rules in assessing the need for computed tomography in patients with mild traumatic brain injury. JAMA Netw Open. 2022;5:e221302.
Sjögren M, Blomberg M, Jonsson M, Wahlund LO, Edman A, Lind K, Rosengren L, Blennow K, Wallin A. Neurofilament protein in cerebrospinal fluid: a marker of white matter changes. J Neurosci Res. 2001;66:510–6.
Gaiottino J, Norgren N, Dobson R, Topping J, Nissim A, Malaspina A, Bestwick JP, Monsch AU, Regeniter A, Lindberg RL, et al. Increased neurofilament light chain blood levels in neurodegenerative neurological diseases. PLoS One. 2013;8:e75091.
Ashton NJ, Janelidze S, Al Khleifat A, Leuzy A, van der Ende EL, Karikari TK, Benedet AL, Pascoal TA, Lleó A, Parnetti L, et al. A multicentre validation study of the diagnostic value of plasma neurofilament light. Nat Commun. 2021;12:3400.
Ashton NJ, Suárez-Calvet M, Karikari TK, Lantero-Rodriguez J, Snellman A, Sauer M, Simrén J, Minguillon C, Fauria K, Blennow K, Zetterberg H. Effects of pre-analytical procedures on blood biomarkers for Alzheimer’s pathophysiology, glial activation, and neurodegeneration. Alzheimers Dement (Amst). 2021;13:e12168.
O’Connell GC, Alder ML, Webel AR, Moore SM. Neuro biomarker levels measured with high-sensitivity digital ELISA differ between serum and plasma. Bioanalysis. 2019;11:2087–94.
Kac PR, Gonzalez-Ortiz F, Simrén J, Dewit N, Vanmechelen E, Zetterberg H, Blennow K, Ashton NJ, Karikari TK. Diagnostic value of serum versus plasma phospho-tau for Alzheimer’s disease. Alzheimers Res Ther. 2022;14:65.
Jonaitis EM, Zetterberg H, Koscik RL, Betthauser TJ, Van Hulle CA, Hogan K, Hegge L, Kollmorgen G, Suridjan I, Gleason CE, et al. Crosswalk study on blood collection-tube types for Alzheimer’s disease biomarkers. Alzheimers Dement. 2022;14:e12266.
Google Scholar
Gonzalez-Ortiz F, Dias A, Turton M, Magalhães R, Kac PR, Correia M, Harrison P, Zetterberg H, Maia LF, Blennow K, Karikari TK. Preanalytical stability of plasma/serum brain-derived tau. Alzheimers Dement. 2023;19(10):4764–70.
Gonzalez-Ortiz F, Ferreira PCL, González-Escalante A, Montoliu-Gaya L, Ortiz-Romero P, Kac PR, Turton M, Kvartsberg H, Ashton NJ, Zetterberg H, et al. A novel ultrasensitive assay for plasma p-tau217: performance in individuals with subjective cognitive decline and early Alzheimer’s disease. Alzheimers Dement. 2023. https://doi.org/10.1002/alz.13525 .
Tumani H, Hegen H. CSF albumin: albumin CSF/serum ratio (marker for blood-CSF barrier function). 2015. pp. 111–114.
Mayo Clinic Test Catalog. https://www.mayocliniclabs.com/en/test-catalog .
Bowen RA, Remaley AT. Interferences from blood collection tube components on clinical chemistry assays. Biochem Med (Zagreb). 2014;24:31–44.
Rózga M, Bittner T, Batrla R, Karl J. Preanalytical sample handling recommendations for Alzheimer’s disease plasma biomarkers. Alzheimers Dement (Amst). 2019;11:291–300.
van Lierop Z, Verberk IMW, van Uffelen KWJ, Koel-Simmelink MJA, In ‘t Veld L, Killestein J, Teunissen CE. Pre-analytical stability of serum biomarkers for neurological disease: neurofilament-light, glial fibrillary acidic protein and contactin-1. Clin Chem Lab Med. 2022;60:842–50.
Jiang L, Ding X, Wang W, Yang X, Li T, Lei P. Head-to-head comparison of different blood collecting tubes for quantification of Alzheimer’s disease biomarkers in plasma. Biomolecules. 2022;12:1194.
Walsh G. Difficult peripheral venous access: recognizing and managing the patient at risk. J Assoc Vasc Access. 2008;13:198–203.
Walter M, Wiltfang J, Vogelgsang J. Pre-analytical sampling and storage conditions of amyloid-β peptides in venous and capillary blood. J Alzheimers Dis. 2020;78:529–35.
Lombardi V, Carassiti D, Giovannoni G, Lu CH, Adiutori R, Malaspina A. The potential of neurofilaments analysis using dry-blood and plasma spots. Sci Rep. 2020;10:97.
Simrén J, Ashton NJ, Blennow K, Zetterberg H. Blood neurofilament light in remote settings: alternative protocols to support sample collection in challenging pre-analytical conditions. Alzheimers Dement. 2021;13:e12145.
Meyer P-F, Ashton NJ, Karikari TK, Strikwerda-Brown C, Köbe T, Gonneaud J, Pichet Binette A, Ozlen H, Yakoub Y, Simrén J, et al. Plasma p-tau231, p-tau181, PET biomarkers, and cognitive change in older adults. Ann Neurol. 2022;91:548–60.
Brum WS, Ashton NJ, Simrén J, di Molfetta G, Karikari TK, Benedet AL, Zimmer ER, Lantero-Rodriguez J, Montoliu-Gaya L, Jeromin A, et al. Biological variation estimates of Alzheimer’s disease plasma biomarkers in healthy individuals. Alzheimers Dement. 2024;20:1284–97.
Heyer NJ, Derzon JH, Winges L, Shaw C, Mass D, Snyder SR, Epner P, Nichols JH, Gayken JA, Ernst D, Liebow EB. Effectiveness of practices to reduce blood sample hemolysis in EDs: a laboratory medicine best practices systematic review and meta-analysis. Clin Biochem. 2012;45:1012–32.
Dugan L, Leech L, Speroni KG, Corriher J. Factors affecting hemolysis rates in blood samples drawn from newly placed IV sites in the emergency department. J Emerg Nurs. 2005;31:338–45.
Bowen RA, Hortin GL, Csako G, Otañez OH, Remaley AT. Impact of blood collection devices on clinical chemistry assays. Clin Biochem. 2010;43:4–25.
Bowen RA, Sattayapiwat A, Gounden V, Remaley AT. Blood collection tube-related alterations in analyte concentrations in quality control material and serum specimens. Clin Biochem. 2014;47:150–7.
Schrapp A, Mory C, Duflot T, Pereira T, Imbert L, Lamoureux F. The right blood collection tube for therapeutic drug monitoring and toxicology screening procedures: standard tubes, gel or mechanical separator? Clin Chim Acta. 2019;488:196–201.
Lewczuk P, Beck G, Esselmann H, Bruckmoser R, Zimmermann RD, Fiszer M, Bibl M, Maler JM, Kornhuber J, Wiltfang J. Effect of sample collection tubes on cerebrospinal fluid concentrations of tau proteins and amyloid β peptides. Clin Chem. 2006;52:332–4.
Perret-Liaudet A, Pelpel M, Tholance Y, Dumont B, Vanderstichele H, Zorzi W, ElMoualij B, Schraen S, Moreaud O, Gabelle A, et al. Cerebrospinal fluid collection tubes: a critical issue for Alzheimer disease diagnosis. Clin Chem. 2012;58:787–9.
Bjerke M, Portelius E, Minthon L, Wallin A, Anckarsäter H, Anckarsäter R, Andreasen N, Zetterberg H, Andreasson U, Blennow K. Confounding factors influencing amyloid Beta concentration in cerebrospinal fluid. Int J Alzheimers Dis. 2010;2010:986310.
Pica-Mendez AM, Tanen M, Dallob A, Tanaka W, Laterza OF. Nonspecific binding of Aβ42 to polypropylene tubes and the effect of Tween-20. Clin Chim Acta. 1833;2010:411.
Toombs J, Paterson RW, Schott JM, Zetterberg H. Amyloid-beta 42 adsorption following serial tube transfer. Alzheimers Res Ther. 2014;6:5.
Lachno DR, Vanderstichele H, De Groote G, Kostanjevecki V, De Meyer G, Siemers ER, Willey MB, Bourdage JS, Konrad RJ, Dean RA. The influence of matrix type, diurnal rhythm and sample collection and processing on the measurement of plasma β-amyloid isoforms using the INNO-BIA plasma Aβ forms multiplex assay. J Nutr Health Aging. 2009;13:220–5.
(CLSI). CaLSI: collection, transport, and processing of blood specimens for testing plasma-based coagulation assays and molecular hemostasis assays; approved guideline—fourth edition. CLSI document H21–A4. Wayne: Clinical and Laboratory Standards Institute; 2017.
Reichstein E. The importance of preanalytical factors in immunodiagnostic testing. EJIFCC. 2003;14:124–7.
Timms JF, Arslan-Low E, Gentry-Maharaj A, Luo Z, T’Jampens D, Podust VN, Ford J, Fung ET, Gammerman A, Jacobs I, Menon U. Preanalytic influence of sample handling on SELDI-TOF serum protein profiles. Clin Chem. 2007;53:645–56.
Sunde AL, Alsnes IV, Aarsland D, Ashton NJ, Tovar-Rios DA, De Santis G, Blennow K, Zetterberg H, Kjosavik SR. Preanalytical stability of plasma biomarkers for Alzheimer’s disease pathology. Alzheimers Dement. 2023;15:e12439.
Lippi G, Blanckaert N, Bonini P, Green S, Kitchen S, Palicka V, Vassault AJ, Plebani M. Haemolysis: an overview of the leading cause of unsuitable specimens in clinical laboratories. Clin Chem Lab Med. 2008;46:764–72.
Keshavan A, Heslegrave A, Zetterberg H, Schott JM. Stability of blood-based biomarkers of Alzheimer’s disease over multiple freeze-thaw cycles. Alzheimers Dement. 2018;10:448–51.
Lierop ZYGJv, Verberk IMW, Uffelen KWJv, Koel-Simmelink MJA, Veld Lit, Killestein J, Teunissen CE. Pre-analytical stability of serum biomarkers for neurological disease: neurofilament-light, glial fibrillary acidic protein and contactin-1. Clin Chem Lab Med. 2022;60:842–850.
Liu HC, Chiu MJ, Lin CH, Yang SY. Stability of plasma amyloid-β 1–40, amyloid-β 1–42, and total tau protein over repeated freeze/thaw cycles. Dement Geriatr Cogn Dis Extra. 2020;10:46–55.
Murphy BM, Swarts S, Mueller BM, van der Geer P, Manning MC, Fitchmun MI. Protein instability following transport or storage on dry ice. Nat Methods. 2013;10:278–9.
Schindler SE, Sutphen CL, Teunissen C, McCue LM, Morris JC, Holtzman DM, Mulder SD, Scheltens P, Xiong C, Fagan AM. Upward drift in cerebrospinal fluid amyloid β 42 assay values for more than 10 years. Alzheimers Dement. 2018;14:62–70.
Kang M, Ragan BG, Park JH. Issues in outcomes research: an overview of randomization techniques for clinical trials. J Athl Train. 2008;43:215–21.
Boulo S, Kuhlmann J, Andreasson U, Brix B, Venkataraman I, Herbst V, Rutz S, Manuilova E, Vandijck M, Dekeyser F, et al. First amyloid β1-42 certified reference material for re-calibrating commercial immunoassays. Alzheimers Dement. 2020;16:1493–503.
Download references
Acknowledgements
R01 AG064877 (MIK), P30 AG066468 (University of Pittsburgh ADRC, OIL as PI), R01 MH116046 (RAS, JK), P01 AG14449 and P01 AG025204 (MDI). HZ is a Wallenberg Scholar supported by grants from the Swedish Research Council (#2022-01018 and #2019-02397), the European Union’s Horizon Europe research and innovation programme under grant agreement No 101053962, Swedish State Support for Clinical Research (#ALFGBG-71320), the Alzheimer Drug Discovery Foundation (ADDF), USA (#201809-2016862), the AD Strategic Fund and the Alzheimer’s Association (#ADSF-21-831376-C, #ADSF-21-831381-C, and #ADSF-21-831377-C), the Bluefield Project, the Olav Thon Foundation, the Erling-Persson Family Foundation, Stiftelsen för Gamla Tjänarinnor, Hjärnfonden, Sweden (#FO2022-0270), the European Union’s Horizon 2020 research and innovation programme under the Marie Skłodowska-Curie grant agreement No 860197 (MIRIADE), the European Union Joint Programme – Neurodegenerative Disease Research (JPND2021-00694), the National Institute for Health and Care Research University College London Hospitals Biomedical Research Centre, and the UK Dementia Research Institute at UCL (UKDRI-1003). KB is supported by the Swedish Research Council (#2017-00915 and #2022-00732), Hjärnfonden, Sweden (#FO2017-0243 and #ALZ2022-0006), and the Swedish state under the agreement between the Swedish government and the County Councils, the ALF-agreement (#ALFGBG-715986 and #ALFGBG-965240). TKK was supported by the NIH (R01 AG083874, U24 AG082930, P30 AG066468, RF1 AG052525-01A1, R01 AG053952-05, R37 AG023651-17, RF1 AG025516-12A1, R01 AG073267-02, R01 AG075336-01, R01 AG072641-02, P01 AG025204-16), the Swedish Research Council (Vetenskåpradet; #2021-03244), the Alzheimer’s Association (#AARF-21-850325), the Swedish Alzheimer Foundation (Alzheimerfonden), the Aina (Ann) Wallströms and Mary-Ann Sjöbloms stiftelsen, and the Emil och Wera Cornells stiftelsen.
Author information
Authors and affiliations.
Department of Psychiatry, School of Medicine, University of Pittsburgh, 3811 O’Hara Street, Pittsburgh, PA, 15213, USA
Xuemei Zeng, Anuradha Sehrawat, Jihui Lee, Tara K. Lafferty, Robert A. Sweet, Dana L. Tudorascu, William E. Klunk, Milos D. Ikonomovic, Anne D. Cohen, Victor L. Villemagne, Tharick A. Pascoal & Thomas K. Karikari
Department of Chemistry, University of Pittsburgh, Pittsburgh, PA, 15213, USA
Department of Pathology, School of Medicine, University of Pittsburgh, Pittsburgh, PA, 15213, USA
Julia Kofler
Department of Neurology, School of Medicine, University of Pittsburgh, Pittsburgh, PA, 15213, USA
Sarah B. Berman, Robert A. Sweet, Milos D. Ikonomovic, Beth E. Snitz, Tharick A. Pascoal & Oscar I. Lopez
Geriatric Research Education and Clinical Center, VA Pittsburgh HS, Pittsburgh, PA, USA
Milos D. Ikonomovic
Department of Psychiatry and Neurochemistry, Institute of Neuroscience and Physiology, The Sahlgrenska Academy, University of Gothenburg, Mölndal, Sweden
Anna Pfister, Henrik Zetterberg, Kaj Blennow & Thomas K. Karikari
Clinical Neurochemistry Laboratory, Sahlgrenska University Hospital, Mölndal, Sweden
Anna Pfister, Henrik Zetterberg & Kaj Blennow
Department of Neurodegenerative Disease, UCL Queen Square Institute of Neurology, London, UK
Henrik Zetterberg
UK Dementia Research Institute at UCL, London, UK
Hong Kong Center for Neurodegenerative Diseases, Hong Kong, China
Department of Medicine, School of Medicine and Public Health, University of Wisconsin-Madison, Madison, WI, USA
Department of Human Genetics, School of Public Health, University of Pittsburgh, Pittsburgh, PA, 15213, USA
M. llyas Kamboh
You can also search for this author in PubMed Google Scholar
Contributions
XZ and TKK contributed to the conception and design of the review. XZ, YC, AS and TKL prepared the first draft of the manuscript, which was critically revised by all authors. All authors contributed to and approved the final version of the manuscript.
Authors’ information
Corresponding author.
Correspondence to Thomas K. Karikari .
Ethics declarations
Ethics approval and consent to participate.
Not applicable.
Consent for publication
Competing interests.
MDI has received research funding from GE Healthcare and Avid Radiopharmaceuticals. HZ has served at scientific advisory boards and/or as a consultant for Abbvie, Acumen, Alector, Alzinova, ALZPath, Annexon, Apellis, Artery Therapeutics, AZTherapies, CogRx, Denali, Eisai, Nervgen, Novo Nordisk, Optoceutics, Passage Bio, Pinteon Therapeutics, Prothena, Red Abbey Labs, reMYND, Roche, Samumed, Siemens Healthineers, Triplet Therapeutics, and Wave, has given lectures in symposia sponsored by Cellectricon, Fujirebio, Alzecure, Biogen, and Roche, and is a co-founder of Brain Biomarker Solutions in Gothenburg AB (BBS), which is a part of the GU Ventures Incubator Program (outside submitted work). KB has served as a consultant and at advisory boards for Acumen, ALZPath, BioArctic, Biogen, Eisai, Lilly, Moleac Pte. Ltd, Novartis, Ono Pharma, Prothena, Roche Diagnostics, and Siemens Healthineers; has served at data monitoring committees for Julius Clinical and Novartis; has given lectures, produced educational materials and participated in educational programs for AC Immune, Biogen, Celdara Medical, Eisai and Roche Diagnostics; and is a co-founder of Brain Biomarker Solutions in Gothenburg AB (BBS), which is a part of the GU Ventures Incubator Program, outside the work presented in this paper. The other authors declare no competing interests.
Additional information
Publisher’s note.
Springer Nature remains neutral with regard to jurisdictional claims in published maps and institutional affiliations.
Supplementary Information
Additional file 1..
Step-by-step blood specimen collection procedures.
Additional file 2.
Simulation datasets used to generate the plots in Fig. 4 .
Rights and permissions
Open Access This article is licensed under a Creative Commons Attribution 4.0 International License, which permits use, sharing, adaptation, distribution and reproduction in any medium or format, as long as you give appropriate credit to the original author(s) and the source, provide a link to the Creative Commons licence, and indicate if changes were made. The images or other third party material in this article are included in the article's Creative Commons licence, unless indicated otherwise in a credit line to the material. If material is not included in the article's Creative Commons licence and your intended use is not permitted by statutory regulation or exceeds the permitted use, you will need to obtain permission directly from the copyright holder. To view a copy of this licence, visit http://creativecommons.org/licenses/by/4.0/ . The Creative Commons Public Domain Dedication waiver ( http://creativecommons.org/publicdomain/zero/1.0/ ) applies to the data made available in this article, unless otherwise stated in a credit line to the data.
Reprints and permissions
About this article
Cite this article.
Zeng, X., Chen, Y., Sehrawat, A. et al. Alzheimer blood biomarkers: practical guidelines for study design, sample collection, processing, biobanking, measurement and result reporting. Mol Neurodegeneration 19 , 40 (2024). https://doi.org/10.1186/s13024-024-00711-1
Download citation
Received : 25 July 2023
Accepted : 13 February 2024
Published : 15 May 2024
DOI : https://doi.org/10.1186/s13024-024-00711-1
Share this article
Anyone you share the following link with will be able to read this content:
Sorry, a shareable link is not currently available for this article.
Provided by the Springer Nature SharedIt content-sharing initiative
- Blood biomarker
- Standardization
- Preanalytical factors
- Neurodegeneration
Molecular Neurodegeneration
ISSN: 1750-1326
- Submission enquiries: Access here and click Contact Us
- General enquiries: [email protected]
Thank you for visiting nature.com. You are using a browser version with limited support for CSS. To obtain the best experience, we recommend you use a more up to date browser (or turn off compatibility mode in Internet Explorer). In the meantime, to ensure continued support, we are displaying the site without styles and JavaScript.
- View all journals
- My Account Login
- Explore content
- About the journal
- Publish with us
- Sign up for alerts
- Open access
- Published: 21 May 2024
Investigating nutrient biomarkers of healthy brain aging: a multimodal brain imaging study
- Christopher E. Zwilling ORCID: orcid.org/0000-0002-2873-0115 1 , 2 ,
- Jisheng Wu ORCID: orcid.org/0009-0003-6000-453X 3 , 4 , 5 &
- Aron K. Barbey ORCID: orcid.org/0000-0002-6092-0912 1 , 2 , 3 , 4 , 5 , 6
npj Aging volume 10 , Article number: 27 ( 2024 ) Cite this article
436 Accesses
237 Altmetric
Metrics details
- Cognitive ageing
The emerging field of Nutritional Cognitive Neuroscience aims to uncover specific foods and nutrients that promote healthy brain aging. Central to this effort is the discovery of nutrient profiles that can be targeted in nutritional interventions designed to promote brain health with respect to multimodal neuroimaging measures of brain structure, function, and metabolism. The present study therefore conducted one of the largest and most comprehensive nutrient biomarker studies examining multimodal neuroimaging measures of brain health within a sample of 100 older adults. To assess brain health, a comprehensive battery of well-established cognitive and brain imaging measures was administered, along with 13 blood-based biomarkers of diet and nutrition. The findings of this study revealed distinct patterns of aging, categorized into two phenotypes of brain health based on hierarchical clustering. One phenotype demonstrated an accelerated rate of aging, while the other exhibited slower-than-expected aging. A t-test analysis of dietary biomarkers that distinguished these phenotypes revealed a nutrient profile with higher concentrations of specific fatty acids, antioxidants, and vitamins. Study participants with this nutrient profile demonstrated better cognitive scores and delayed brain aging, as determined by a t-test of the means. Notably, participant characteristics such as demographics, fitness levels, and anthropometrics did not account for the observed differences in brain aging. Therefore, the nutrient pattern identified by the present study motivates the design of neuroscience-guided dietary interventions to promote healthy brain aging.
Similar content being viewed by others
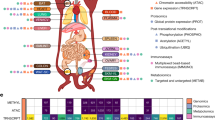
Temporal dynamics of the multi-omic response to endurance exercise training
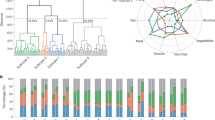
Associations of dietary patterns with brain health from behavioral, neuroimaging, biochemical and genetic analyses
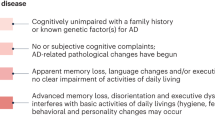
Alzheimer’s disease risk reduction in clinical practice: a priority in the emerging field of preventive neurology
Introduction.
Accumulating evidence in Nutritional Cognitive Neuroscience indicates that diet and nutrition may benefit the aging brain (for a review, see ref. 1 ). A recent review of the literature surveyed 52 studies comprising more than 21,000 participants and found that dietary markers of the Mediterranean Diet were associated with healthy brain aging, as measured by MRI indices of structural and functional connectivity 2 . Despite the promise of these findings, questions remain about the causal effects of diet and nutrition on brain health and their role in age-related neurobiological decline; for example, whether elements of the Mediterranean Diet, such as polyunsaturated fatty acids (PUFAs), may limit the reduction in white matter volume with age. The potential benefits of the Mediterranean Diet may result from its focus on nutrient classes that have known functional relationships with the brain. For example, fatty acids, including monounsaturated, polyunsaturated, and saturated fatty acids, are necessary for structural brain integrity and development, cellular energy metabolism, and neurotransmission and neuromodulation 3 . Indeed, randomized controlled trials (RCTs) examining the effects of fatty acids on brain health typically observe improvements in brain function, white matter integrity, and gray matter volume 4 , 5 , 6 , 7 . Notably, however, RCTs that investigate the effects of fatty acids on cognitive performance (without additional measures of brain health) demonstrate mixed results, with positive 8 or null findings 9 . In addition to fatty acids, the Mediterranean Diet includes antioxidants (i.e., vitamins, flavonoids, and carotenoids), which are known to reduce oxidative stress and therefore to benefit brain health 10 , 11 . RCTs examining the effects of antioxidants on the aging brain demonstrate benefits in cerebral blood flow and for measures of functional brain connectivity (e.g., functional brain network integration 12 ). Evidence further suggests that antioxidants may have favorable effects on episodic memory, although these findings do not extend to all forms of memory affected by aging 7 , 13
More broadly, a large association study of ~75,000 participants revealed that greater consumption of antioxidants was associated with a lower chance of developing subjective cognitive impairment in late life 14 . Finally, research also suggests that choline, an essential nutrient that promotes structural brain integrity, cellular energy metabolism, and neurotransmission, may improve multiple facets of cognition in older adults 15 . Taken together, these findings suggest that nutrition may support and enhance cognitive function and brain health, especially in healthy older adults.
The potential for nutritional interventions to promote healthy brain function is particularly significant given the well-established effects of aging on cognitive performance and brain health 16 , 17 , 18 , 19 , 20 , 21 . Senescence is accompanied by age-related neurodegeneration in gray and white matter structures and an increase in ventricular space 22 . White matter fiber integrity declines with age, as indexed by decreased fractional anisotropy and increases in axial, radial, and mean diffusivity 23 . Concentrations of metabolic markers of neuronal integrity, measured by magnetic resonance spectroscopy (MRS), also decline with age 22 . Advancing age is associated with smaller cerebral volume, likely due to cortical neuronal degeneration and synaptic density reduction, in addition to reduced cortical thickness and surface area 24 , 25 . The observed changes in the aging brain are also known to affect cognitive function, producing declines in cognitive control, fluid intelligence, processing speed, and memory 21 , 26 , 27 , 28 , 29 .
Age-related changes in brain health are known to vary within the population, reflecting individual differences in the onset, duration, and severity of age-related neurological symptoms 19 , 30 , 31 . Thus, chronological age alone does not fully explain the complex trajectory of brain health in late life. Indeed, recent evidence demonstrates that although structural MRI measures can predict chronological age, there are often deviations in the predicted and observed aging trajectory, such that accelerated aging results in a brain that is older than expected, whereas delayed aging results in a brain that is younger than predicted 32 , 33 .
Although the literature on healthy aging has identified important risk factors that accelerate brain aging, much less is known about preventative factors that reduce the severity of neurobiological disease in late life 34 . Our research therefore sought to identify nutrient biomarker patterns that are associated with Accelerated versus Delayed Brain Aging, with an interest in guiding the development of nutritional interventions designed to promote healthy brain aging. Specifically, the present study was motivated by three primary aims. First, we sought to identify distinct phenotypes of Accelerated versus Delayed Brain Aging within a sample of 100 healthy older adults. Brain imaging measures were acquired from a comprehensive battery of over 100 neuroimaging markers of brain health, including measures of brain structure (i.e., volumetrics and white matter tracts), functional brain connectivity, and brain metabolites, as measured by MRS. Second, using a well-validated neuropsychological test battery, we compared performance on measures of intelligence, executive function, and memory in the Accelerated versus Delayed Brain Aging phenotypes. Finally, we investigated whether the observed phenotypes captured distinct nutrient biomarker profiles, with a focus on nutrients that are known to have favorable effects on cognitive function and brain health from the Mediterranean Diet (i.e., fatty acids, antioxidants, and vitamins).
We predicted that phenotypes of Accelerated versus Delayed Brain Aging would emerge, given well-established individual differences in brain aging trajectories. We also predicted that these distinct phenotypes would embody differences in cognitive function manifested by the observed differences in brain aging. Finally, our predictions about the role of nutrition in healthy brain aging were guided by findings to suggest that specific nutrients may benefit brain health, including poly- and mono-unsaturated fatty acids, vitamins, antioxidants, and carotenoids. Thus, by combining advances in Nutritional Cognitive Neuroscience—nutrient biomarkers of diet, multimodal brain imaging, and statistical modeling of brain aging—this interdisciplinary study aimed to identify nutrient profiles associated with Accelerated versus Delayed Brain Aging and to establish nutritional targets for future interventions designed to promote brain health.
Brain health phenotypes
A total of 139 variables of brain health were collected in the study, including measures of structure, function, and metabolism (Table 1 ). Brain volumes were measured separately for the left and right hemispheres for the Structural Regions listed in Table 1 , for a total of 68 regions. Total white matter integrity was measured for each of the Structural Regions in Table 1 , for a total of 34 tracts. Functional connectivity was assessed using four graph theory metrics on each of 8 brain networks, for a total of 32 measures. Finally, three metabolite concentrations were measured within each brain region.
Hierarchical clustering of all 139 brain measures collected in the study revealed two distinct phenotypes of brain health: Accelerated and Delayed Aging. Hierarchical clustering uses a similarity metric that identifies individuals who are most like one another across all brain measures. The two phenotypes identified by hierarchical clustering indicate that, across all study participants, one group is similar with less optimal brain health while the other group is similar with more optimal brain health. The dendrogram is presented in Supplementary Fig. 1 . The average value for the two phenotypes for each brain imaging domain from Table 1 —structure, metabolism, and functional connectivity—is listed in Table 2 . Relative to individuals with Accelerated Aging, those with Delayed Aging have larger brain volumes (0.48 vs 0.43), increased white matter integrity (0.61 vs 0.49), increased concentrations of brain metabolites (0.444 vs 0.441), increased functional connectivity for the whole brain (0.51 vs 0.45) and small world propensity (0.52 vs 0.43). Network functional connectivity was greater for individuals with Accelerated Aging relative to those with Delayed Aging for strength (0.57 vs 0.45), local efficiency (0.52 vs 0.47) and global efficiency (0.55 vs 0.43). Supplementary Figs. 2 , 3 and 4 present heatmaps of the values for each participant for each network or region of all brain measurements presented in Table 1 .
Brain age and brain health phenotypes
Brain Age (BA) was estimated for both brain health phenotypes. Individuals in the Accelerated Aging phenotype have an average BA of 65.1 whereas those in the Delayed Aging have an average BA of 59.7. This difference of 5.4 BA years is significant ( t statistic = 2.66, p value = 0.010). Chronological Age (CA) and BA are presented in Fig. 1 for both brain phenotypes. Many individuals in the Accelerated Aging phenotype have BAs closer to their CAs whereas many individuals in the Delayed Aging phenotype have BAs less than their CAs. Correlations between BA were computed with multiple brain modalities. BA was negatively associated with brain volumes ( r = −0.19, p value = 0.058), white matter tracts ( r = −0.37, p value = 0.00014), brain metabolites ( r = −0.25, p value = 0.017) and functional connectivity measures ( r = −0.19, p value = 0.063). These robust negative correlations suggest that a young BA is associated with the Delayed Brain Aging phenotype, indicating multiple brain modalities can predict BA specifically and brain health generally.
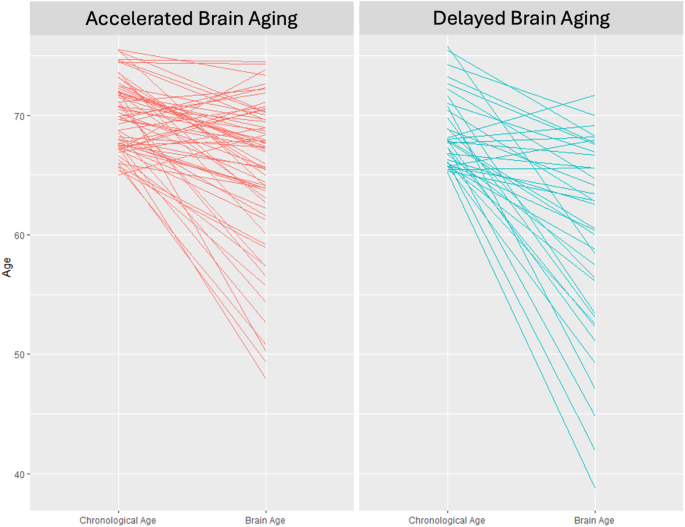
Chronological age (left side of each panel) versus brain age (right side of each panel) for the accelerated (left panel) and delayed (right panel) brain aging phenotypes.
Brain health and cognition
The study results established robust differences in brain structure, function, and metabolism between the two phenotypes of brain health. Furthermore, these phenotypes were inversely correlated with BA, where the individuals with Accelerated Aging have an Old BA and those with Delayed Aging have a Young BA. We next examined how differences in Delayed and Accelerated Brain Aging phenotypes map onto cognition. We assessed measures of intelligence (WASI), executive function (DKEFS), and memory (WMS). Individuals with Delayed Brain Aging outperformed those with Accelerated Brain Aging for all cognitive tests (see Fig. 2 ). Two scores for the Delayed Brain Aging Phenotype, reaction time (DKEFS Trails 5) and response errors (DKEFS Trails Errors), are negative and smaller than the Accelerated Brain Aging Phenotype, reflecting better cognitive performance for the Delayed Brain Aging Phenotype. A t test comparing the means between the Delayed and Accelerated Brain Aging groups is significant (p-value < 0.05) for tests of general and fluid intelligence (WASI_FSIQ4 and WASI_PRI), executive function (DKEFS_Trails.1) and memory (WMS_IMI). Supplementary Table 1 includes the scaled scores for all 15 cognitive tests.
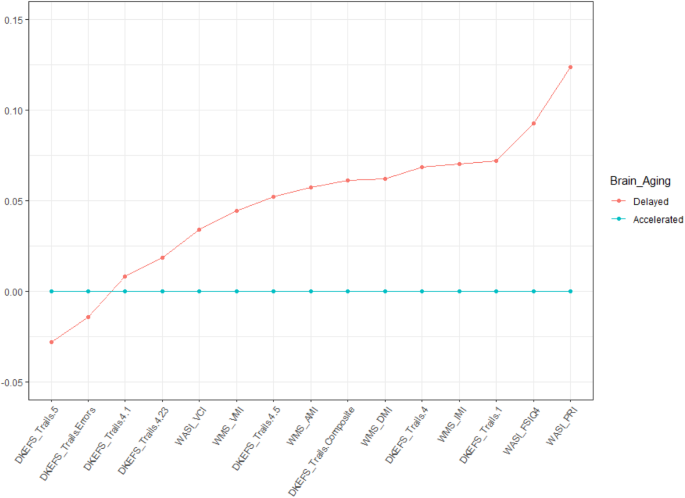
The scores for Accelerated Brain Aging were set to a baseline of 0 for each cognitive measure and the Delayed Brain Aging group is expressed as a difference relative to that baseline, with values further from the baseline reflecting a larger difference. All cognitive scores were scaled between 0 and 1.
Nutrient profile of healthy brain aging
Having established two distinct phenotypes of brain aging derived from measures of brain structure, function, and metabolism, and based on a comprehensive cognitive battery, we lastly determined the nutritional profile of the Delayed Brain Aging phenotype (illustrated in Table 3 ). We examined the nutritional status of study participants using blood-based biomarkers. The nutrient biomarker profile of individuals in the Delayed Brain Aging phenotype was characterized by greater concentrations of 13 key nutrients compared to the Accelerated Aging phenotype, as determined by a t-test comparing the group means. Notably, the observed nutrient profile encompassed several nutrient categories important for brain health, including monounsaturated fatty acids (C18:1n-7 and C20:1n-9), ω-3 polyunsaturated fatty acids (C18:3n-3 and C20:5n-3, commonly known as ALA and EPA, respectively), ω-6 polyunsaturated fatty acids (C22:2n-6 and C20:2n-6), one long-chain saturated fatty acid (C24:0), the carotenoids lutein and zeaxanthin, and vitamin E and choline (see Supplementary Table 2 ). Nutrients that did not differ between the Accelerated versus Delayed Brain Aging phenotypes include Vitamins A, B2, B6, B12, D and E, carotenoids lycopene and carotene, short and medium chain saturated fatty acids (i.e., C10:0 to C22:0), and some PUFAs (e.g., C18:4n-3 and C22:4n-6). The coefficient of variability and intraclass correlation coefficient for the blood biomarkers in Table 3 are presented in Supplementary Table 3 .
Additionally, to rule out the possibility that other covariates contributed to brain aging differences between the phenotypes, we investigated multiple measures of demographics, anthropometrics, and physical fitness collected in the study. None of these variables differed between the two phenotypes using a t-test of the means, suggesting these factors do not explain the observed differences in brain aging (see Supplementary Table 4 ). Finally, following standard conventions, we controlled for BMI, sex, income, and education by including these factors as covariates in the analysis.
Nutritional Cognitive Neuroscience aims to identify specific foods and nutrients that promote healthy brain aging. Central to this effort is the discovery of nutrient profiles that can be targeted in nutritional interventions designed to promote brain health with respect to multimodal neuroimaging measures of brain structure, function, and metabolism. The present study advances four primary conclusions pursuant to this goal.
First, we provide evidence for a multimodal characterization of healthy brain aging, classified according to neuroimaging measures associated with Delayed or Accelerated Brain Aging. Specifically, relative to Accelerated Brain Aging, older adults with Delayed Brain Aging exhibited: (1) larger brain volumes, (2) better structural DTI integrity across 34 brain regions, (3) better functional connectivity for whole brain and network-level measures of local and global efficiency, and for small world propensity, and (4) greater concentrations of the brain metabolites choline, creatine, and NAA. These findings are notable given that prior research has focused primarily on a single brain imaging modality, limiting the nature and scope of conclusions drawn about the role of diet and nutrition in healthy brain aging.
Second, Delayed Brain Aging was inversely correlated with neuroimaging biomarkers of BA, demonstrating that a younger BA is associated with favorable brain health outcomes with respect to measures of brain structure, function, and metabolism 35 .
Third, scores on cognitive assessments of intelligence, executive function, and memory were higher for older adults with Delayed Brain Aging compared to those with Accelerated Brain Aging. These results are consistently supported by findings from the cognitive neuroscience of aging, namely that older adults with larger brain volumes, white matter tracts with greater integrity, and more efficient functional connectivity also demonstrate better cognitive performance 26 , 27 , 28 .
Fourth, the discovered nutrient profile for healthy brain aging was not derived from dietary questionnaires, as is typically employed in nutritional epidemiology, but from nutrient biomarkers which accurately reflect the concentrations of nutrients from the diet 36 , 37 . The observed nutrient profile is both broad, including fatty acids, carotenoids, and vitamins, and specific, identifying the amount and type of specific nutrients in each category.
Finally, the current study identified a nutrient profile related to healthy brain aging and a clinically relevant neuroimaging biomarker of BA. Although nutrition represents an established risk factor for age-related neurological disease 34 , the potential benefits of nutrition for promoting brain health are less well understood. Thus, the nutrient pattern identified by the present study motivates the design of neuroscience-guided dietary interventions to promote healthy brain aging. We now review the primary elements of the observed nutritional pattern and the mechanisms of action that have been proposed in the nutritional sciences to explain their benefits on cognitive and brain health.
Fatty acids
In our study, the fatty acid nutritional profile of individuals in the Delayed Brain Aging phenotype includes increased concentrations of EPA, ALA, docosadienoic acid, and eicosadienoic acid, all of which are known to reduce inflammation. Inflammation makes the vascular blood–brain barrier more permeable to cytokines, and chemokines, which interfere with neuronal and glial well-being and interrupt brain homeostasis 38 , 39 , 40 . Accumulating evidence has linked increased inflammation to decline in brain structure and function, cognitive decline, and increased risk of dementia 41 , 42 , 43 . We review the relationship between inflammation and each of these fatty acids in turn below.
Eicosanoids, signaling molecules responsible for cellular functions regulating inflammation and the central nervous system, derive from competing metabolic pathways that begin with three different 20-carbon fatty acids: arachidonic acid (20:4 ω-6, AA), eicosapentaenoic acid (20:5 ω-3, EPA), and di-homo γ linolenic acid (20:3 ω-6, DGLA) 44 . The ω-6 AA pathway promotes inflammation whereas the ω-3 DGLA and EPA pathways are less inflammatory, biologically inert, or even anti-inflammatory. Moreover, the three pathways compete for the same enzymes, rate-limiting molecules, transport, and acylation pathways. Hence, the greater the presence of anti-inflammatory generating ω-3 EPA in the diet, as is observed in our nutrient profile of Delayed Brain Aging, the fewer inflammatory eicosanoids will be generated by the AA pathway.
Another nutrient in the Delayed Brain Aging phenotype related to EPA and beneficial inflammation is α-linolenic acid or ALA. ALA is one of two essential fatty acids. EPA is obtained through diet or endogenously produced, via a metabolic pathway beginning with ALA. ALA converts to EPA with 10–20% efficiency 45 . Thus, greater concentrations of ALA in the diet, as is the case for individuals with healthy brain aging, can theoretically yield more of the beneficial EPA to compete with the inflammatory AA cascade. Excellent dietary sources of EPA and ALA include fish and shellfish, flaxseed, hemp seed, olive oil, soya oil, canola oil, chia seeds, pumpkin seeds, sunflower seeds, leafy vegetables, and walnuts.
The DGLA pathway is another beneficial competitor to the AA cascade, resulting in less severe inflammation or even anti-inflammatory metabolites. Two other nutrients in the profile of Delayed Brain Aging, eicosadienoic and docosadienoic acids, are closely related to the DGLA pathway. Eicosadienoic acid is the direct precursor of DGLA while docosadienoic acid is the immediate elongation product of DGLA. These two fatty acids have antioxidant abilities and anti-inflammatory properties meeting or exceeding those of DHA 46 . They also exhibit inhibitory activity against inflammation-causing enzymes by exerting similar physiological effects as over the counter non-steroidal anti-inflammatory drugs (e.g., ibuprofen) that block the COX-I and COX-II enzymes responsible for inflammation and pain 47 .
Saturated fatty acids are traditionally viewed as unhealthy 48 . However recent research suggests some long-chain fatty acids, with more than 20 carbon atoms, may confer health benefits, with evidence suggesting that they are associated with lower risk of coronary heart disease and type 2 diabetes, and may promote healthy aging 49 . Our nutrient profile of Delayed Brain Aging includes one very long chain saturated fatty acid, lignoceric acid (C24.0). Peanuts, macadamia nuts, and certain seed oils are excellent sources of lignoceric acid 49 . A recent study demonstrated that higher concentrations of long-chain fatty acids in plasma in mid-life resulted in reduced cognitive decline in a test of verbal fluency 20 years later 50 . Another study examined the concentration of lignoceric acid in brain tissue and discovered that females without cognitive impairment exhibited a larger concentration of C24.0 compared to females who developed Alzheimer’s disease. These findings provide a rationale for suggesting that long-chain saturated fatty acids, and lignoceric acid specifically, are important biomarkers of cognitive and brain health.
The final two fatty acids discovered in our nutrient profile of Delayed Brain Aging, vaccenic and gondoic acids, are both mono-unsaturated fatty acids, or MUFAs. MUFAs, which are common in olive oil and the Mediterranean Diet, are known to support brain and cognitive health 51 . Both vaccenic and gondoic acid have robust antioxidant activities 47 . Vaccenic acid is the primary type of fat from dairy products, such as milk, butter, and yogurt. Increasing consumption of dairy products increases the concentration of vaccenic acid in plasma 52 . The importance of vaccenic acid for brain health may lie in its metabolic conversion to conjugated linoleic acid, or CLA 53 . CLA is incorporated and metabolized into brain tissue, which further extends its anti-neuroinflammatory properties 54 . Nervonic acid is the predominant fatty acid in the white matter tissue of humans and one of its metabolic precursors is gondoic acid 55 . Based on these findings, it is possible that increased dietary concentrations of vaccenic and gondoic acids may enhance white matter brain integrity, although future research in Nutritional Cognitive Neuroscience is needed to clarify the precise role of these MUFAs in brain and cognitive health.
Carotenoids
Three different carotenoids, phytopigments that give many fruits and vegetables their characteristic color, figure prominently in the nutritional profile of Delayed Brain Aging. Carotenoid-rich foods include spinach, kale, corn, bell peppers (red, green, or yellow), tomatoes, watermelon, grapefruit, cantaloupe, broccoli, and carrots. Carotenoids have known benefits to cognitive and brain health, as demonstrated by studies that examine their effects on brain structure, brain network function, and memory 7 , 56 , 57 , 58 . Carotenoids accumulate in the retina of the eye and in the brain, and greater consumption of carotenoids increases their concentration in these tissues 59 . Carotenoids are known to benefit the brain because of their antioxidant properties. The brain is particularly vulnerable to oxidative stress due to its high lipid concentrations and high energy requirements 60 .
Vitamin E and choline were identified as important nutrients that promote cognitive and brain health in the Delayed Brain Aging phenotype. Multiple studies, including RCTs, have shown that high concentrations of Vitamin E in plasma are associated with better cognitive performance in healthy populations, aging populations, and Alzheimer disease patients 56 , 61 . Vitamin E’s efficacy in mitigating cognitive decline is likely through its antioxidant properties and its ability to aid in the transporting of fatty acids 62 . A recent RCT demonstrated that supplementation of Vitamin E, along with ω-3 fatty acids and carotenoids, improves performance on tests of working memory 57 . These findings are consistent with the results of the present study, which observed higher scores on tests of intelligence and memory within the Delayed Brain Aging phenotype. Notably, intelligence and memory are supported by multiple cortical regions (e.g., prefrontal, cingulate, and parietal cortices) and networks (e.g., frontoparietal network, the default mode network, and the salience network) 63 . Within the Delayed Brain Aging phenotype, we observed that these regions and networks demonstrated superior performance compared to the Accelerated Brain Aging phenotype based on measures of cortical volume and functional brain network efficiency, respectively. Excellent dietary sources of Vitamin E include nuts, seeds, and vegetable oils while significant amounts also come from green leafy vegetables and fortified cereals.
Choline, an essential B-vitamin-like nutrient, is also in the nutrient profile of Delayed Brain Aging. Choline plays at least two critical functions for cognitive and brain health: it is a necessary precursor for phosphatidylcholine, the predominant lipid in cell membranes and it is required for the synthesis and release of acetylcholine, a critical neurotransmitter 64 . Furthermore, brain white matter tracts and brain volume, which are enhanced in the Delayed Brain Aging phenotype, critically depend on choline for their cellular structure and integrity. Choline benefits both executive function and memory 15 , 64 . Excellent dietary sources of choline include animal-based proteins such as meat, poultry, fish, and eggs, while cruciferous vegetables and certain beans are also rich in choline.
Overall, there is strong evidence to support the nutrient profile underlying the Delayed Brain Aging phenotype in promoting cognitive and brain health. Many of the biochemical pathways underlying fatty acid synthesis and metabolism are well-known; but the implications of those competing pathways for cognitive and brain health, which importantly depend on the nutrients available from the diet, are only beginning to be understood. Moreover, future research should examine the differential impact of nutrition on different brain regions and networks, as certain nutrients may be important for different regions of the brain whereas other nutrients are required by the brain globally. Applying methods from Nutritional Cognitive Neuroscience, future RCTs should systematically investigate the effects of specific nutrient profiles on the structural integrity and functional efficiency of specific cortical regions and networks (e.g., combining nutrient biomarker analysis with MRI measures of local and global brain network operations). The current results provide evidence that some metabolic pathways (e.g., the DGLA pathway compared to the AA pathway) may yield more optimal brain and cognitive outcomes. Furthermore, the observed metabolic pathways that are less optimal for cognitive and brain health often result in higher levels of inflammation. Carotenoids and vitamins identified in the current study that benefit cognitive and brain health, such as lutein, choline, and Vitamin E, require regular consumption to have their beneficial effect. Importantly, these nutrients may accumulate preferentially in certain brain regions or networks, motivating an investigation of the selectivity of nutrition for promoting the health and function of specific brain regions and cortical networks.
Limitations
While some of the nutrients observed in the present study have solid molecular mechanisms to help explain their role in cognitive and brain health, other nutrients are less well understood. Research on long-chain saturated fatty acids and MUFAs (vaccenic acid and gondoic acid) have several preliminary studies suggesting their benefits on cognitive and brain health, but more research is needed to establish the precise mechanisms by which they exert an effect. Another limitation of the present study concerns the cross-sectional study design, sample size, and Caucasian participants. The Delayed Brain Aging phenotype identifies a set of nutrients that longitudinal and randomized controlled trials should target in future studies to determine their effects on cognitive and brain aging. Other statistical tools, such as canonical correlation analysis and structural equation modeling, may also be applied to draw new insights about the associations between brain aging and nutrition. The results of the present study need to be examined in non-Caucasian participants to assess the generality of findings. Moreover, while the current study examined brain health applying measures of brain structure, function, and metabolism, future research should also seek to understand how diet and nutrition effect the trajectory of brain aging within each of these measures. Additionally, the nutrient profile of Delayed Brain Aging identified in the current study does not imply they are the only nutrients that matter for brain health. Clearly, the brain needs many nutrients for healthy functioning, including amino acids, multiple B vitamins, ω-3 and ω-6 polyunsaturated fatty acids, monounsaturated fatty acids, choline, Vitamins C and D, and minerals like iron, zinc, and magnesium 65 . The nutrient profile identified in the current study differentiates Delayed Brain Aging from Accelerated Brain Aging. Finally, while the nutrient profiles discovered here suggest a basis for future testing of dietary interventions for optimal brain health, additional studies are needed to further establish and validate the present findings. It will also be important to build large-scale studies and research consortia to investigate the relationship between alternate measures of dietary intake and nutritional status, examining the reliability and validity of nutrient biomarkers, food frequency questionnaires, and their respective merits and limitations 66 , 67 .
The present study identified a specific profile of nutrients that may promote healthy brain aging, motivating further research to establish and validate these findings in the context of a randomized controlled trial. By building upon the observed findings, future research can inform the development of more effective, targeted dietary interventions that apply methods in Nutritional Cognitive Neuroscience. We believe this approach holds promise for the development of dietary strategies to support cognitive function and brain health in the aging population.
This cross-sectional study enrolled 100 healthy elderly adults from the Illinois Brain Aging Study cohort, a sample of community-dwelling Caucasian men and women aged 65–75 years. Participants were neurologically healthy and did not have evidence of cognitive impairment, as determined by a score of lower than 26 on the Mini-Mental State Examination 68 . Participants with mild cognitive impairment, dementia, a psychiatric illness within the last three years, a stroke within the past twelve months, cancer in the last three years, an inability to complete study activities, prior involvement in cognitive training or dietary intervention studies, or contraindications for magnetic resonance imaging (MRI) were excluded. All participants were right-handed with normal, or corrected to normal, vision.
Standard protocol approval and patient consent
In accordance with the University of Illinois and Carle Foundation Hospital Institutional Review Boards, informed consent was obtained from all participants in this study.
Nutrient biomarker acquisition and analysis
Fasting plasma was collected from each participant between 7:00 AM and 12:00 noon Central Time. Nutrient biomarkers were assayed, comprising three general classes of nutrients: fatty acids, carotenoids, and vitamins. Ethylenediaminetetraacetic acid (EDTA) plasma carotenoids and tocopherols were analyzed by high-performance liquid chromatography with a photodiode array detector (HPLC-PDA) using UV detection 69 . Plasma lipids were measured with gas chromatography using flame ionization and peaks of interest were identified by comparison to authentic fatty acid standards 70 . Vitamins were measured by a chemiluminescent immunometric assay or after extraction by radioimmunoassay 71 , 72 .
MRI data acquisition and processing
All data were collected on a Siemens Magnetom 3T Trio scanner using a 32-channel head coil in the MRI Laboratory of the Beckman Institute Biomedical Imaging Center at the University of Illinois.
MRI data acquisition
A high-resolution multi-echo T1-weighted magnetization prepared gradient-echo structural image was acquired for each participant (0.9 mm isotropic, TR: 1900 ms, TI: 900 ms, TE = 2.32 ms, with GRAPPA and an acceleration factor of 2). The functional neuroimaging data were acquired using an accelerated gradient-echo echoplanar imaging sequence sensitive to blood oxygenation level dependent (BOLD) contrast (2.5 × 2.5 × 3.0 mm voxel size, 38 slices with 10% slice gap, TR = 2000 ms, TE = 25 ms, FOV = 230 mm, 90° flip angle, 7 min acquisition time). During the resting-state fMRI scan, participants were shown a white crosshair on a black background viewed on an LCD monitor through a head coil-mounted mirror. Participants were instructed to lie still, focus on the visually presented crosshair, and to keep their eyes open 73 .
MRI data preprocessing
All MRI data processing was performed using FSL tools available in Functional Magnetic Resonance Imaging of the Brain (FMRIB) Software Library version 5.0. The high-resolution T1 Magnetization-Prepared Rapid Gradient-Echo (MPRAGE) was extracted using the Brain Extraction Tool 74 . FMRIB’s Automated Segmentation Tool 75 was applied to delineate gray matter, white matter, and cerebral spinal fluid (CSF) voxels. The resting-state fMRI data were pre-processed using the FSL FMRI Preprocessing and Model-Based Analysis (FEAT) analysis tool 76 , 77 . Pre-processing entailed: slice timing correction, motion correction, spatial smoothing (3 mm full width at half maximum kernel), nuisance signal regression (described below), standard fMRI temporal bandpass filtering (0.009–0.1 Hz, linear registration of functional images to structural images, and non-linear registration of structural images to the MNI152 brain template (2 mm isotropic voxel resolution).
Nuisance variables were modeled via General Linear Modeling (GLM) analyses to remove spurious correlations, noise introduced by head motion, and variables of no interest. These included head motion correction parameters (using the extended 12 motion parameters estimated in FEAT preprocessing), modeling of individual volume motion outliers estimated using DVARS (outliers flagged using the boxplot cutoff 1.5 × interquartile range 76 ), and averaging of mean white matter and cerebrospinal fluid signals across all voxels identified from the segmentation of the high resolution MPRAGE. The fully preprocessed resting-state fMRI data comprised the residual obtained from fitting these nuisance variables in the GLM framework. The residuals were transformed into normalized MNI152 space and re-sampled to 4 mm isotropic voxels to reduce computational complexity in post data processing for network analysis.
Brain volumetrics
Cortical reconstruction was performed with the Freesurfer image analysis software 78 . For this analysis, all the cortical gray matter volumes provided by the Freesurfer parcellation were examined. This included 68 regions throughout the frontal, parietal, temporal, and occipital lobes. Volumetric measures were adjusted for intracranial volume and sex using a regression model. The adjusted values were then used in further statistical analyses.
Volumetric analysis was performed on data from a 3D high-resolution (0.9 mm isotropic) T1-weighted scan using MPRAGE acquisition. Cortical reconstruction was performed with the Freesurfer image analysis suite, which is documented and freely available for download online ( http://surfer.nmr.mgh . harvard.edu/ ). All cortical reconstructions were manually checked for accuracy, as recommended by the software developers. The volumetric analyses focused on gray matter volume in the temporal cortex provided by Freesurfer parcellation. Regions of interest included the superior temporal cortex, middle temporal cortex, inferior temporal cortex, banks of the superior temporal sulcus, fusiform cortex, transverse temporal cortex, entorhinal cortex, temporal pole, and parahippocampal cortex.
Diffusion tractography imaging (DTI)
Whole brain diffusion tensor imaging was acquired with the following parameters: FOV = 240 × 240 mm; 72 slices, slice thickness = 2 mm; TE = 98 ms; TR = 10,000 ms; in-plane resolution = 1.875 × 1.875 mm; diffusion encoding directions = 30; b = 0 s/mm 2 and 1000 s/mm 2 . Data were processed using the University of Oxford’s Center for FMRIB Software Library (FSL) release 5.0 79 diffusion toolbox 80 , 81 . Eddy current correction was accomplished using the eddy correction tool and a diffusion tensor model was fit in each voxel using the DTIFIT tool, which generates fractional anisotropy (FA) values in every voxel. FA images were further processed using the FSL tract-based spatial statistics 82 toolbox, which projects each subjects’ FA data onto a mean white matter skeleton, representing the white-matter tracts common to all subjects. Mean FA within the white matter skeleton for specific regions of interest were calculated for each subject using the JHU ICBM DTI-81 atlas 83 .
Graph theory metrics of brain efficiency
The efficiency of brain network function was examined by investigating the small-world organization 84 of seven well-established intrinsic connectivity networks of the brain 85 . A small-word organization represents the optimal balance of local and global network efficiency, providing a parsimonious neural architecture that supports high local clustering (local efficiency) and short average path length (global efficiency). The procedure for computing small-world propensity is presented below.
First, the mean fMRI BOLD time series was extracted from subjects’ gray matter voxels using the Craddock parcellated brain atlas as a mask 86 . This parcellation of 200 regions provided whole-brain coverage and sufficiently high spatial resolution for conducting network analysis. A subject-wise functional connectivity matrix reflecting pairwise Pearson correlations between the mean BOLD time series signals obtained from nodes defined by the Craddock atlas was then computed and Fisher’s Z-transformed to achieve normality. These were standardized to Z-scores through multiplication with their standard deviation approximated as σ = 1/√( n − 3), where n is the number of time points corresponding to the BOLD signal 87 . A Bonferroni-corrected statistical Z-threshold was applied to identify significant positive correlations ( p < 0.05) within each subject’s whole-brain functional connectivity matrix derived from Craddock’s 800 parcellation atlas 88 , 89 . The thresholded Z-scores were rescaled to represent connection weights ranging from 0 to 1. Based on these positive connection weights, weighted connectivity matrices representing functional connectivity between nodes representative of seven intrinsic connectivity networks were obtained for each subject. The seven intrinsic connectivity network maps—visual, somatosensory, limbic, default mode, dorsal attention, ventral attention and frontoparietal—are at https://surfer.nmr.mgh.harvard.edu/fswiki/CorticalParcellation_Yeo2011 .
We then examined small-world propensity within the rescaled connectivity matrices derived for each of the seven intrinsic connectivity networks. Small world propensity Φ is calculated as the fractional deviation between a network’s clustering coefficient, C obs , and characteristic path length, L obs , from both lattice ( C latt , L latt ) and random ( C rand , L rand ) networks constructed with the same number of nodes and the same degree distribution:
The ratios Δ C and Δ L represent the fractional deviation of the metric ( C obs or L obs ) from its respective null model (a lattice or random network).
Magnetic resonance spectroscopy (MRS)
Metabolite concentration from MRS was determined using the procedure in ref. 90 . The anatomical scan was used to position a single voxel spectroscopy (SVS) scan in the parietal cortex extending into posterior cingulate cortex (voxel size: (20 mm), TR: 3000 ms, TE: 30 ms, 40 averages, BW: 2000 Hz, vector size: 1024). The voxel straddled the midline, including equal portions of the right and left hemispheres. Weak water suppression was employed, and six regional saturation bands were placed around the voxel to reduce contamination from subcutaneous fat. An additional scan was performed without water suppression to aid with quantification. Immediately following the spectroscopy acquisition, a T2-weighted overlay scan was performed with the same center location and orientation as the spectroscopy scan (TR = 5000 ms, TE = 84 ms, slice thickness 2 mm with 0.5 mm of spacing, FOV: 240 × 240 mm, 128 × 128 matrix size, GRAPPA acceleration factor: 2, 35 slices).
Metabolite quantitation was performed using tissue water as a reference. Water-scaled spectra were analyzed using LCModel software (Version 6.3-1H). No correction was performed to account for relaxation of metabolite signal. Because NAA and NAAG are difficult to differentiate, here we analyze the combined concentration of NAA + NAAG, labeled herein as NAAt with a peak appearing at 2.02 ppm.
Accurate water scaling requires corrections for the tissue composition of the voxel. Using the high-resolution structural scan, we calculated the volume fractions of gray matter (GM), white matter (WM), and CSF within each voxel using Matlab scripts (MathWorks, Natick, MA) that called functions from SPM8 (Wellcome Trust Centre for Neuroimaging). First, we segmented the MPRAGE using SPM8 to obtain tissue probability maps of GM, WM and CSF. We then created a mask in the space of the T2 overlay corresponding to the location of the spectroscopy scan. This mask has the same center and orientation as the T2 overlay but higher resolution (0.5 × 0.5 × 0.5 mm). We then registered the MPRAGE to the T2 overlay. The rotations and translations required for the registration were then applied to the tissue probability masks. We resliced the tissue probability maps into the space of the mask, and used the mask to calculate the volume fractions of GM, WM, and CSF within the volume of the spectroscopy voxel. These tissue fractions were later used to statistically correct NAAt for tissue volume-fraction dependencies.
Brain age (BA)
BA was derived using Brain-Age Regression Analysis and Computation Utility Software, or BARACUS, using T1-weighted structural images 33 , 91 .
Cognitive assessments
Neuropsychological tests investigating multiple facets of memory, executive function, and intelligence were administered. Our battery included the Wechsler Adult Intelligence Scale (WAIS 92 ), the trail-making test from the Delis-Kaplan Executive Function System (DKEFS 93 ), and the Wechsler Memory Systems (WMS 94 ).
Memory was measured by the Wechsler Memory Scale—Fourth Edition (WMS-IV) Older Adult Battery 94 . This assessment measured memory by way of four indices: Auditory Memory Index, Visual Memory Index, Immediate Memory Index, and Delayed Memory Index. The Auditory Memory Index indicates a participant’s ability to remember orally presented information. The Visual Memory Index indicates a participant’s ability to remember visually presented information. The Immediate Memory Index indicates a participant’s ability to recall visually and orally presented information immediately after it is presented. The Delayed Memory Index indicates a participant’s ability to recall and recognize visually- and orally-presented information after a 20 to 30-min delay. Participants’ raw scores on each subtest were converted to normalized scaled scores and subsequently combined into indices. Z-scores for each index were calculated and then averaged to create a composite memory score 95 .
Executive function
Executive functions were measured by the Delis–Kaplan Executive Function System (D–KEFS) Trail Making Test 93 . This assessment yields a measure of the executive functions that can be isolated from underlying skills, including visual scanning, number sequencing, letter sequencing, and motor speed. In this task, participants alternate between multiple task goals (either number or letter sequencing), which elicits a specific component of the executive functions known as cognitive flexibility. The reported results from the D-KEFS Trail Making Test assess cognitive flexibility while controlling for number and letter sequencing trials and therefore provide a measure of cognitive flexibility that is not confounded by underlying cognitive abilities (i.e., number and letter sequencing) required by the task.
Intelligence
General intelligence was measured by the Wechsler Abbreviated Scale of Intelligence– second edition (WASI-II 92 ). Per the scoring guidelines, this assessment measured general intelligence by way of an estimated intelligence quotient score, derived from fluid and crystallized test scores which, in turn, were derived from four subtests: a block design subtest, a matrix reasoning subtest, a vocabulary subtest, and a similarities subtest. In the block design subtest, participants were asked to reproduce pictured designs using specifically designed blocks as quickly and accurately as possible. In the matrix reasoning subtest, participants were asked to complete a matrix or serial reasoning problem by selecting the missing section from five response items. In the vocabulary subtest, participants were asked to verbally define vocabulary words (i.e., What does lamp mean?) that became progressively more challenging. In the similarities subtest, participants were asked to relate pairs of concepts (i.e., How are a cow and bear alike?) that became progressively more challenging. Per scoring guidelines, subjects’ raw scores were converted to standardized scores and combined into an estimated intelligence quotient score, which provided a measure of general intelligence.
Demographics, anthropometrics, and fitness
Demographics, including sex, education, and income, were collected via questionnaire responses provided by the participants. Anthropometric measures of weight, height, hip, and waist circumference were assessed by the study coordinator at the first study visit. Several measures of physical fitness were also collected or determined. Body Mass Index (BMI) was calculated from measured height and weight. Resting Heart Rate (RHR) was measured by the study coordinator during the participant’s first visit to the lab. Physical activity and the metabolic equivalent of VO 2 were assessed via a published questionnaire and required BMI and RHR as inputs 96 .
Analysis overview
First, individual differences in brain health were determined from the multimodal neuroimaging features using hierarchical clustering. Individuals with similar brain health were grouped together. Second, brain aging trajectories were computed for everyone and for each brain health group. Third, we examined cognitive aging differences within each brain health group. Fourth, a nutrient profile for the healthy brain and cognitive aging group was determined. Finally, lifestyle factors other than diet were examined to determine their role in brain and cognitive aging.
Statistical analyses
All statistical analyses were conducted in R Studio Version 2022.2.3.492 97 using the R statistical computing software environment Version 4.0.3 98 . Covariates with the weakest inter-correlations associated with age-related cognitive decline (age, gender, education, income, and Body Mass Index) were included as predictors in a separate regression model for each outcome measure of nutrition, brain, and cognition. Residualization was performed using the Frisch-Waugh-Lowell theorem. The net result of residualization is to remove the potential effects of covariates on the outcome. The residuals from each model were normally transformed using Tukey’s Ladder of Powers 99 and then [0,1] scaled. This process also had the effect of transforming outlier values to a normal range and resulting in a range of 0 to 1 for all variables. Hierarchical clustering, using the complete linkage method, was used to cluster individuals according to similarity of their brain features and it is not sensitive to multicollinearity. T tests of the mean were used to assess the magnitude and significance of differences between phenotypes.
Reporting summary
Further information on research design is available in the Nature Research Reporting Summary linked to this article.
Data availability
The individual de-identified participant data can be made available upon request.
Code availability
Codes used for this study are available upon reasonable request from the authors.
Zamroziewicz, M. & Barbey, A. Nutritional cognitive neuroscience: innovations for healthy brain aging. Front. Neurosci. 10 , 240 (2016).
Article PubMed PubMed Central Google Scholar
Jensen, D., Leoni, V., Klein-Flügge, M., Ebmeier, K. & Suri, S. Associations of dietary markers with brain volume and connectivity: a systematic review of MRI studies. Ageing Res. Rev. 70 , 101360 (2021).
Article CAS PubMed Google Scholar
Tracey, T., Steyn, F., Wolvetang, E. & Ngo, S. Neuronal lipid metabolism: multiple pathways driving functional outcomes in health and disease. Front. Mol. Neurosci. 11 , 10 (2018).
Köbe, T., Witte, A., Schnelle, A., Lesemann, A. & Fabian, S. Combined omega-3 fatty acids, aerobic exercise and cognitive stimulation prevents decline in gray matter volume of the frontal, parietal and cingulate cortex in patients with mild cognitive impairment. NeuroImage 131 , 226–238 (2016).
Article PubMed Google Scholar
Witte, A., Kerti, L., Hermannstädter, H., Fiebach, J. & Schreiber, S. Long-chain omega-3 fatty acids improve brain function and structure in older adults. Cereb. Cortex 24 , 3059–3068 (2014).
Jernerén, F., Elshorbagy, A., Oulhaj, A., Smith, S. & Refsum, H. Brain atrophy in cognitively impaired elderly: the importance of long-chain ω-3 fatty acids and B vitamin status in a randomized controlled trial. Am. J. Clin. Nutr. 102 , 215–221 (2015).
Sueyasu, T., Yasumoto, K., Tokuda, H., Kaneda, Y. & Obata, H. Effects of long-chain polyunsaturated fatty acids in combination with lutein and zeaxanthin on episodic memory in healthy older adults. Nutrients 15 , 2825 (2023).
Article CAS PubMed PubMed Central Google Scholar
Zwilling, C., Strang, A., Anderson, E., Jurcsisn, J. & Johnson, E. Enhanced physical and cognitive performance in active duty Airmen: evidence from a randomized multimodal physical fitness and nutritional intervention. Nat. Sci. Rep. 10 , 17826 (2020).
CAS Google Scholar
Mazereeuw, G., Lanctôt, K., Chau, S., Swardfager, W. & Herrmann, N. Effects of ω-3 fatty acids on cognitive performance: a meta-analysis. Neurobiol. Aging 33 , e17–e29 (2012).
Article Google Scholar
Franzoni, F. et al. Oxidative stress and cognitive decline: the neuroprotective role of natural antioxidants. Front. Neurosci. 15 , 729–757 (2021).
Terracina, S., Petrella, C., Francati, S., Lucarelli, M. & Barbato, C. Antioxidant intervention to improve cognition in the aging brain: the example of hydroxytyrosol and resveratrol. Int. J. Mol. Sci. 23 , 156–174 (2022).
Lindbergh, C., Lv, J., Zhao, Y., Mewborn, C. & Puente, A. The effects of lutein and zeaxanthin on resting state functional connectivity in older Caucasian adults: a randomized controlled trial. Brain Imaging Behav. 14 , 668–681 (2020).
Sloan, R., Wall, M. & Yeung, L. Insights into the role of diet and dietary flavanols in cognitive aging: results of a randomized controlled trial. Sci. Rep. 11 , 3837 (2021).
Yeh, T., Yuan, C., Ascherio, A., Rosner, B. & Willett, W. Long-term dietary flavonoid intake and subjective cognitive decline in US men and women. Neurology 97 , e1041–e1056 (2021).
CAS PubMed PubMed Central Google Scholar
Nakazaki, E., Mah, E., Sanoshy, K., Citrolo, D. & Watanabe, F. Citicoline and memory function in healthy older adults: a randomized, double-blind, placebo-controlled clinical trial. J. Nutr. 151 , 2153–2160 (2021).
López-Otín, C., Blasco, M., Partridge, L., Serrano, M. & Kroemer, G. The hallmarks of aging. Cell 153 , 1194–1217 (2013).
Kirkwood, T. Understanding the odd science of aging. Cell 120 , 437–447 (2005).
Kirkwood, T. B. A systematic look at an old problem. Nature 451 , 644–647 (2008).
Wrigglesworth, J., Ward, P., Harding, I., Nilaweera, D. & Wu, Z. Factors associated with brain ageing—a systematic review. BMC Neurolol. 21 , 312 (2021).
Bethlehem, R., Seidlitz, J., White, S. & Vogel, J. Brain charts for the human lifespan. Nature 604 , 525–533 (2022).
Harada, C., Natelson Love, M. & Triebel, K. Normal cognitive aging. Clin. Geriatr. Med. 29 , 737–752 (2013).
Raz, N. & Rodrigue, K. Differential aging of the brain: patterns, cognitive correlates and modifiers. Neurosci. Biobehav. Rev. 30 , 730–748 (2006).
Sexton, C., Walhovd, K., Storsve, A., Tamnes, C. & Westlye, L. Accelerated changes in white matter microstructure during aging: a longitudinal diffusion tensor imaging study. J. Neurosci. 34 , 15425–15436 (2014).
Lockhart, S. & DeCarli, C. Structural imaging measures of brain aging. Neuropsychol. Rev. 24 , 271–289 (2014).
Fjell, A., McEvoy, L., Holland, D., Dale, A. & Walhovd, K. Alzheimer’s Disease Neuroimaging Initiative Brain changes in older adults at very low risk for Alzheimer’s disease. J. Neurosci. 33 , 8237–8242 (2013).
Park, D. & Reuter-Lorenz, P. The adaptive brain: aging and neurocognitive scaffolding. Annu. Rev. Psychol. 60 , 173–196 (2009).
Salthouse, T. What and when of cognitive aging. Curr. Direc. Psychol. Sci. 13 , 140–144 (2004).
IJ, D., Corley, J., Gow, A., Harris, S. & Houlihan, L. Age-associated cognitive decline. Br. Med. Bull. 92 , 135–152 (2009).
Craik, F. I., Salthouse, T. A. The Handbook of Aging and Cogntion, 1st edn (Psychology Press, New York City, 2008).
Mattay, V., Goldberg, T., Sambataro, F. & Weinberger, D. Neurobiology of cognitive aging: insights from imaging genetics. Biol. Psychol. 79 , 9–22 (2008).
Nyberg, L. & Pudas, S. Successful memory aging. Annu. Rev. Psychol. 70 , 219–243 (2019).
Franke, K., Ziegler, G., Klöppel, S. & Gaser, C. Alzheimer’s Disease Neuroimaging Initiative Estimating the age of healthy subjects from T1-weighted MRI scans using kernel methods: exploring the influence of various parameters. Neuroimage 50 , 883–892 (2010).
Liem, F. Predicting brain-age from multimodal imaging data captures cognitive impairment. Neuroimage 148 , 179–188 (2017).
Franke, K. & Gaser, C. Ten years of brainAGE as a neuroimaging biomarker of brain aging: what insights have we gained? Front. Neurol 10 , 789 (2019).
Talukdar, T., Zwilling, C. & Barbey, A. Integrating nutrient biomarkers, cognitive function, and structural MRI data to build multivariate phenotypes of healthy aging. J. Nutr. 153 , 1338–1346 (2023).
Zamroziewicz, M., Paul, E. J., Zwilling, C. E. & Barbey, A. K. Determinants of fluid intelligence in healthy aging: omega-3 polyunsaturated fatty acid status and frontoparietal cortex structure. Nutr. Neurosci. 21 , 570–579 (2018).
Zamroziewicz, M., Paul, E., Zwilling, C. & Barbey, A. Predictors of memory in healthy aging: polyunsaturated fatty acid balance and fornix white matter integrity. Aging Dis. 8 , 372–383 (2017).
Galea, I. The blood-brain barrier in systemic infection and inflammation. Cell Mol. Immunol. 18 , 2489–2501 (2021).
Sankowski, R., Mader, S. & Valdés-Ferrer, S. Systemic inflammation and the brain: novel roles of genetic, molecular, and environmental cues as drivers of neurodegeneration. Front. Cell Neurosci. 9 , 29 (2015).
Sun, Y., Koyama, Y. & Shimada, S. Inflammation from peripheral organs to the brain: how does systemic inflammation cause neuroinflammation? Front. Aging Neurosci. 14 , 903455 (2022).
Sartori, A., Vance, D., Slater, L. & Crowe, M. The impact of inflammation on cognitive function in older adults: implications for healthcare practice and research. J. Neurosci. Nurs. 44 , 206–217 (2012).
Sæther, L., Ueland, T., Haatveit, B., Maglanoc, L. & Szabo, A. Inflammation and cognition in severe mental illness: patterns of covariation and subgroups. Mol. Psychiatry 28 , 1284–1292 (2023).
Kipinoinen, T., Toppala, S., Rinne, J., Viitanen, M. & Jula, A. Association of midlife inflammatory markers with cognitive performance at 10-year follow-up. Neurology 99 , e2294–e2302 (2022).
Soberman, R. & Christmas, P. The organization and consequences of eicosanoid signaling. J. Clin. Investig. 111 , 1107–1113 (2003).
Goyens, P., Spilker, M., Zock, P., Katan, M. & Mensink, R. Conversion of α-linolenic acid in humans is influenced by the absolute amounts of α-linolenic acid and linoleic acid in the diet and not by their ratio. Am. J. Clin. Nutr. 84 , 44–53 (2006).
Chen, Y., Qiu, X. & Yang, J. Comparing the in vitro antitumor, antioxidant and anti-inflammatory activities between two new very long-chain polyunsaturated fatty acids, docosadienoic acid (DDA) and docosatrienoic acid (DTA), and docosahexaenoic acid (DHA). Nutr. Cancer 73 , 1697–1707 (2021).
Henry, G., Momin, R., Nair, M. & Dewitt, D. Antioxidant and cyclooxygenase activities of fatty acids found in food. J. Agric. Food Chem. 50 , 2231–2234 (2002).
Caballero, B. Ed., Encyclopedia of Human Nutrition in Health Effects of Saturated Fatty Acids , 215–219 (Academic Press, 2013).
Lemaitre, R. & King, I. Very long-chain saturated fatty acids and diabetes and cardiovascular disease. Curr. Opin. Lipidol. 33 , 76–82 (2022).
Li, D., Misialek, J., Jing, M., Tsai, M. & Eckfeldt, J. Plasma phospholipid very-long-chain SFAs in midlife and 20-year cognitive change in the Atherosclerosis Risk in Communities (ARIC): a cohort study. Am. J. Clin. Nutr. 111 , 1252–1258 (2020).
Zamroziewicz, M., Talukdar, M., Zwilling, C. & Barbey, A. Nutritional status, brain network organization, and general intelligence. NeuroImage 161 , 241–250 (2017).
Burdge, G., Tricon, S., Morgan, R., Kliem, K. & Childs, C. Incorporation of cis-9, trans-11 conjugated linoleic acid and vaccenic acid (trans-11 18:1) into plasma and leucocyte lipids in healthy men consuming dairy products naturally enriched in these fatty acids. Br. J. Nutr. 94 , 237–243 (2005).
Field, C., Blewett, H., Proctor, S. & Vine, D. Human health benefits of vaccenic acid. Appl. Physiol. Nutr. Metab. 34 , 979–991 (2009).
Murru, E., Carta, G., Manca, C., Sogos, V. & Pistis, M. Conjugated linoleic acid and brain metabolism: a possible anti-neuroinflammatory role mediated by PPARα activation. Front. Pharmacol. 11 , 587140 (2021).
Fan, Y., Meng, H., Hu, G. & Li, F. Iosynthesis of nervonic acid and perspectives for its production by microalgae and other microorganisms. Appl. Microbiol. Biotechnol. 102 , 3027–3035 (2018).
Zwilling, C., Talukdar, T., Zamroziewicz, M. & Barbey, A. Nutrient biomarker patterns, cognitive function, and fMRI measures of network efficiency in the aging brain. NeuroImage 188 , 239–251 (2019).
Power, R., Nolan, J., Prado-Cabrero, A., Roche, W. & Coen, R. Omega-3 fatty acid, carotenoid and vitamin E supplementation improves working memory in older adults: A randomised clinical trial. Clin Nutr 41 , 405–414 (2022).
Ceravolo, S., Hammond, B., Oliver, W., Clementz, B. & Miller, L. Dietary carotenoids lutein and zeaxanthin change brain activation in older adult participants: a randomized, double-masked, placebo-controlled trial. Mol. Nutr. Food Res. 63 , 15 (2019).
Tanprasertsuk, J., Scott, T., Barbey, A., Barger, K. & Wang, X. Carotenoid-rich brain nutrient pattern is positively correlated with higher cognition and lower depression in the oldest old with no dementia. Front. Nutr. 8 , 704691 (2021).
Lee, K., Cha, M. & Lee, B. Neuroprotective effect of antioxidants in the brain. Int. J. Mol. Sci. 21 , 7152 (2020).
La Fata, G., Weber, P. & Mohajeri, M. Effects of vitamin E on cognitive performance during ageing and in Alzheimer’s disease. Nutrients 6 , 5453–5472 (2014).
Lee, P. & Ulatowski, L. Vitamin E: mechanism of transport and regulation in the CNS. IUBMB Life 71 , 424–429 (2019).
Colom, R., Karama, S., Jung, R. & Haier, R. Human intelligence and brain networks. Front. Psychol. 12 , 489–501 (2010).
Google Scholar
Zamroziewicz, M., Zwilling, C. & Barbey, A. Inferior prefrontal cortex mediates the relationship between phosphatidylcholine and executive functions in healthy, older adults. Front. Aging Neurosci. 8 , 226 (2016).
PubMed PubMed Central Google Scholar
Gómez-Pinilla, F. Brain foods: the effects of nutrients on brain function. Nat. Rev. Neurosci. 9 , 568–578 (2008).
Bowman, G., Shannon, J., Ho, E., Traber, M. & Frei, B. Reliability and validity of food frequency questionnaire and nutrient biomarkers in elders with and without mild cognitive impairment. Alzheimer Dis. Assoc. Disord. 25 , 49–57 (2011).
Fraser, G., Jaceldo-Siegl, K., Henning, S., Fan, J. & Knutsen, S. Biomarkers of dietary intake are correlated with corresponding measures from repeated dietary recalls and food-frequency questionnaires in the adventist health study-2. J. Nutr. 146 , 586–594 (2016).
Folstein, M., Folstein, S. & McHugh, P. Mini-mental state”. A practical method for grading the cognitive state of patients for the clinician. J. Psychiatr. Res. 12 , 189–198 (1975).
Zhimin, X. & Howard, L. R. Analysis of Antioxidant‐Rich Phytochemicals (John Wiley & Sons Ltd, 2012).
Folch, J., Lees, M. & Stanley, G. A simple method for the isolation and purification of total lipids from animal tissues. J. Biol. Chem. 226 , 497–509 (1957).
Babson, A. The Cirrus Immulite automated immunoassay system. J. Clin. Immunoassay 14 , 83–88 (1991).
Hart, G., Furniss, J., Laurie, D. & Durham, S. Measurement of vitamin D status: Background, clinical use, and methodologies. Clin Lab 52 , 335–343 (2006).
CAS PubMed Google Scholar
Van Dijk, K., Hedden, T., Venkataraman, A., Evans, K. & Lazar, S. Intrinsic functional connectivity as a tool for human connectomics: theory, properties, and optimization. J. Neurophysiol. 103 , 297–321 (2010).
Smith, S. Fast robust automated brain extraction. Hum. Brain Mapp. 17 , 143–155 (2002).
Zhang, Y., Brady, M. & Smith, S. Segmentation of brain MR images through a hidden Markov random field model and the expectation-maximization algorithm. IEEE Trans. Med. Imaging 20 , 45–57 (2001).
Jenkinson, M., Beckmann, C., Behrens, T., Woolrich, M. & Smith, S. Fsl. Neuroimage 62 , 782–790 (2012).
Satterthwaite, T., Wolf, D. & Loughead, J. Impact of in-scanner head motion on multiple measures of functional connectivity: relevance for studies of neurodevelopment in youth. Neuroimage 60 , 623–632 (2012).
Reuter, M., Rosas, H. D. & Fischl, B. Highly accurate inverse consistent registration: a robust approach. Neuroimage 53 , 1181–1196 (2010).
Smith, S., Jenkinson, M., Woolrich, M., Beckmann, C. & Behrens, T. Advances in functional and structural MR image analysis and implementation as FSL. Neuroimage 23 , S208–S219 (2004).
Behrens, T. E. et al. Characterization and propagation of uncertainty in diffusion-weighted MR imaging. Magn. Reson. Med. 50 , 1077–1088 (2003).
Behrens, T., Berg, H., Jbabdi, S., Rushworth, M. & Woolrich, M. Probabilistic diffusion tractography with multiple fibre orientations: what can we gain? Neuroimage 34 , 144–155 (2007).
Smith, S., Jenkinson, M., Johansen-Berg, H., Rueckert, D. & Nichols, T. Tract-based spatial statistics: Voxelwise analysis of multi-subject diffusion data. Neuroimage 31 , 1487–1505 (2006).
Oishi, K., Zilles, K., Amunts, K., Faria, A. & Jiang, H. Human brain white matter atlas: identification and assignment of common anatomical structures in superficial white matter. Neuroimage 43 , 447–457 (2008).
Muldoon, S., Bridgeford, E. & Bassett, D. Small-world propensity and weighted brain networks. Sci. Rep. 6 , 22057 (2016).
Yeo, B., Krienen, F. & Sepulcre, J. The organization of the human cerebral cortex is estimated by intrinsic functional connectivity. J. Neurophysiol. 106 , 1125–1165 (2011).
Craddock, R., James, G., Holtzheimer, P., Hu, X. & Mayberg, H. A whole brain fMRI atlas generated via spatially constrained spectral clustering. Hum. Brain Mapp. 33 , 1914–1928 (2012).
Ree, M. Correlation and regression: applications for industrial organizational psychology and management. Organ. Res. Methods 5 , 200–201 (2002).
Fox, M., Zhang, D., Snyder, A. & Raichle, M. The global signal and observed anticorrelated resting state brain networks. J. Neurophysiol. 101 , 3270–3283 (2009).
Murphy, K., Birn, R., Handwerker, D., Jones, T. & Bandettini, P. The impact of global signal regression on resting state correlations: are anti-correlated networks introduced?. Neuroimage 44 , 893–905 (2009).
Paul, E., Larsen, R. & Barbey, A. Dissociable brain biomarkers of fluid intelligence. NeuroImage 137 , 201–211 (2016).
Fischl, B. FreeSurfer. NeuroImage 62 , 774–781 (2012).
Wechsler, D. Wechsler Abbreviated Scale of Intelligence (Psychological Corporation, 1999).
Delis, D. C., Kaplan, E. & Kramer, J. H. Delis-Kaplan Executive Function System (D–KEFS) (The Psychological Corporation, San Antonio, 2001).
Wechsler, D. WMS-IV: Wechsler Memory Scale-fourth Edition (Pearson, San Antonio, 2009).
Siedlecki, K., Honig, L. & Stern, Y. Exploring the structure of a neuropsychological battery across healthy elders and those with questionable dementia and Alzheimer’s disease. Neuropsychology 22 , 400–411 (2009).
Jurca, R., Jackson, A., LaMonte, M., Morrow, J. J. & Blair, S. Assessing cardiorespiratory fitness without performing exercise testing. Am. J. Prev. Med. 29 , 185–193 (2005).
CoreTeam, R Studio: Integrated Development Environment for R (RStudio, PBC, Boston, 2022).
Team, R: A Language and Environment for Statistical Computing (R Foundation for Statistical Computing, Vienna, 2020).
Salvatore, M. rcompanion: Functions to Support Extension Education Program Evaluation , R package version 2.3.25, (2020).
Download references
Acknowledgements
This work was supported by a grant from Abbott Nutrition through the Center for Nutrition, Learning, and Memory at the University of Illinois (ANGC1205; PI: Barbey). The interdisciplinary community and supportive environment within the Decision Neuroscience Laboratory ( https://www.decisionneurosciencelab.org ) proved invaluable in completing this research.
Author information
Authors and affiliations.
Department of Psychology, University of Illinois, Urbana, IL, USA
Christopher E. Zwilling & Aron K. Barbey
Beckman Institute for Advanced Science and Technology, University of Illinois, Urbana, IL, USA
Decision Neuroscience Laboratory, University of Nebraska-Lincoln, Lincoln, NE, USA
Jisheng Wu & Aron K. Barbey
Center for Brain, Biology, and Behavior, University of Nebraska-Lincoln, Lincoln, NE, USA
Department of Psychology, University of Nebraska-Lincoln, Lincoln, NE, USA
Department of Bioengineering, University of Illinois, Urbana, IL, USA
Aron K. Barbey
You can also search for this author in PubMed Google Scholar
Contributions
Project Conception (C.E.Z., J.W., and A.K.B.), Study Design (C.E.Z., J.W., and A.K.B.), Data Analysis (C.E.Z. and J.W.), Manuscript drafting (C.E.Z., J.W., and A.K.B.), Manuscript editing and final review (C.E.Z. and A.K.B.).
Corresponding author
Correspondence to Aron K. Barbey .
Ethics declarations
Competing interests.
The authors declare no competing interests.
Additional information
Publisher’s note Springer Nature remains neutral with regard to jurisdictional claims in published maps and institutional affiliations.
Supplementary information
Supplemental information, reporting summary, rights and permissions.
Open Access This article is licensed under a Creative Commons Attribution 4.0 International License, which permits use, sharing, adaptation, distribution and reproduction in any medium or format, as long as you give appropriate credit to the original author(s) and the source, provide a link to the Creative Commons licence, and indicate if changes were made. The images or other third party material in this article are included in the article’s Creative Commons licence, unless indicated otherwise in a credit line to the material. If material is not included in the article’s Creative Commons licence and your intended use is not permitted by statutory regulation or exceeds the permitted use, you will need to obtain permission directly from the copyright holder. To view a copy of this licence, visit http://creativecommons.org/licenses/by/4.0/ .
Reprints and permissions
About this article
Cite this article.
Zwilling, C.E., Wu, J. & Barbey, A.K. Investigating nutrient biomarkers of healthy brain aging: a multimodal brain imaging study. npj Aging 10 , 27 (2024). https://doi.org/10.1038/s41514-024-00150-8
Download citation
Received : 18 October 2023
Accepted : 15 April 2024
Published : 21 May 2024
DOI : https://doi.org/10.1038/s41514-024-00150-8
Share this article
Anyone you share the following link with will be able to read this content:
Sorry, a shareable link is not currently available for this article.
Provided by the Springer Nature SharedIt content-sharing initiative
Quick links
- Explore articles by subject
- Guide to authors
- Editorial policies
Sign up for the Nature Briefing: Translational Research newsletter — top stories in biotechnology, drug discovery and pharma.

Neuroticism and inflammatory skin diseases: a bidirectional Mendelian randomization study
- ORIGINAL PAPER
- Published: 24 May 2024
- Volume 316 , article number 213 , ( 2024 )
Cite this article
- Charalabos Antonatos 1 ,
- Alexandros Pontikas 1 ,
- Adam Akritidis 1 ,
- Sophia Georgiou 2 ,
- Alexander J. Stratigos 3 ,
- Ileana Afroditi Kleidona 3 ,
- Stamatis Gregoriou 3 ,
- Katerina Grafanaki 2 , 4 &
- Yiannis Vasilopoulos 1
Previous observational studies have linked inflammatory skin diseases with mental health issues and neuroticism. However, the specific impact of neuroticism and its subclusters (i.e. worry, depressed affect, and sensitivity to environmental stress and adversity) on these conditions remains underexplored. In this work, we explored causal associations between common inflammatory skin diseases and neuroticism. We conducted a two-sample, bidirectional Mendelian randomization (MR) analysis using data from genome-wide association studies in psoriasis, atopic dermatitis, neuroticism and relevant genetic subclusters conducted on participants of European ancestry. Corrections for sample overlap were applied where necessary. We found that psoriasis was causally associated with increased levels of worry (odds ratio, 95% confidence intervals: 1.011, 1.006–1.016, P = 3.84 × 10 –6 ) while none of the neuroticism subclusters showed significant association with psoriasis. Sensitivity analyses revealed considerable evidence of directional pleiotropy between psoriasis and neuroticism traits. Conversely, genetic liability to atopic dermatitis did not exhibit any significant association with neuroticism traits. Notably, genetically predicted worry was linked to an elevated risk of atopic dermatitis (odds ratio, 95% confidence intervals: 1.227, 1.067–1.41, P = 3.97 × 10 –3 ). Correction for overlapping samples confirmed the robustness of these results. These findings suggest potential avenues for future interventions aimed at reducing stress and worry among patients with inflammatory skin conditions.
This is a preview of subscription content, log in via an institution to check access.
Access this article
Price includes VAT (Russian Federation)
Instant access to the full article PDF.
Rent this article via DeepDyve
Institutional subscriptions
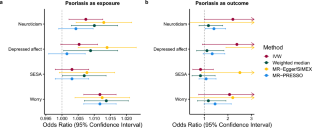
Parisi R, Iskandar IYK, Kontopantelis E et al (2020) National, regional, and worldwide epidemiology of psoriasis: systematic analysis and modelling study. BMJ 369:m1590. https://doi.org/10.1136/bmj.m1590
Article PubMed PubMed Central Google Scholar
Langan SM, Mulick AR, Rutter CE et al (2023) Trends in eczema prevalence in children and adolescents: A Global Asthma Network Phase I Study. Clin Exp Allergy 53:337–352. https://doi.org/10.1111/cea.14276
Article PubMed Central Google Scholar
Yew YW, Kuan AHY, Ge L et al (2020) Psychosocial impact of skin diseases: a population-based study. PLoS ONE 15:e0244765. https://doi.org/10.1371/journal.pone.0244765
Article CAS PubMed PubMed Central Google Scholar
Mento C, Rizzo A, Muscatello MRA et al (2020) Negative emotions in skin disorders: a systematic review. Int J Psychol Res (Medellin) 13:71–86. https://doi.org/10.21500/20112084.4078
Article PubMed Google Scholar
Nagel M, Watanabe K, Stringer S et al (2018) Item-level analyses reveal genetic heterogeneity in neuroticism. Nat Commun 9:905. https://doi.org/10.1038/s41467-018-03242-8
Nagel M, Speed D, van der Sluis S, Østergaard SD (2020) Genome-wide association study of the sensitivity to environmental stress and adversity neuroticism cluster. Acta Psychiatr Scand 141:476–478. https://doi.org/10.1111/acps.13155
Article CAS PubMed Google Scholar
Schonmann Y, Mansfield KE, Hayes JF et al (2020) Atopic eczema in adulthood and risk of depression and anxiety: a population-based cohort study. J Allergy Clin Immunol Pract 8:248-257.e16. https://doi.org/10.1016/j.jaip.2019.08.030
Henderson AD, Adesanya E, Mulick A et al (2023) Common mental health disorders in adults with inflammatory skin conditions: nationwide population-based matched cohort studies in the UK. BMC Med 21:285. https://doi.org/10.1186/s12916-023-02948-x
Wang Y, Wang X, Gu X et al (2023) Evidence for a causal association between psoriasis and psychiatric disorders using a bidirectional Mendelian randomization analysis in up to 902,341 individuals. J Affect Disord 337:27–36. https://doi.org/10.1016/j.jad.2023.05.059
Baurecht H, Welker C, Baumeister S-E et al (2021) Relationship between atopic dermatitis, depression and anxiety: a two-sample Mendelian randomization study. Br J Dermatol 185:781–786. https://doi.org/10.1111/bjd.20092
Rukh G, de Ruijter M, Schiöth HB (2023) Effect of worry, depression, and sensitivity to environmental stress owing to neurotic personality on risk of cardiovascular disease: a Mendelian randomization study. J Pers 91:856–867. https://doi.org/10.1111/jopy.12782
Stuart PE, Tsoi LC, Nair RP et al (2022) Transethnic analysis of psoriasis susceptibility in South Asians and Europeans enhances fine-mapping in the MHC and genomewide. HGG Adv 3:100069. https://doi.org/10.1016/j.xhgg.2021.100069
Budu-Aggrey A, Kilanowski A, Sobczyk MK et al (2023) European and multi-ancestry genome-wide association meta-analysis of atopic dermatitis highlights importance of systemic immune regulation. Nat Commun 14:6172. https://doi.org/10.1038/s41467-023-41180-2
Richmond RC, Sanderson E (2022) Methods and practical considerations for performing Mendelian randomization. Int J Epidemiol 51:2031–2034. https://doi.org/10.1093/ije/dyac166
Article Google Scholar
Verbanck M, Chen C-Y, Neale B, Do R (2018) Detection of widespread horizontal pleiotropy in causal relationships inferred from Mendelian randomization between complex traits and diseases. Nat Genet 50:693–698. https://doi.org/10.1038/s41588-018-0099-7
Bowden J, Del Greco MF, Minelli C et al (2016) Assessing the suitability of summary data for two-sample Mendelian randomization analyses using MR-Egger regression: the role of the I2 statistic. Int J Epidemiol 45:1961–1974. https://doi.org/10.1093/ije/dyw220
Mounier N, Kutalik Z (2023) Bias correction for inverse variance weighting Mendelian randomization. Genet Epidemiol 47:314–331. https://doi.org/10.1002/gepi.22522
Mrowietz U, Sümbül M, Gerdes S (2023) Depression, a major comorbidity of psoriatic disease, is caused by metabolic inflammation. J Eur Acad Dermatol Venereol 37:1731–1738. https://doi.org/10.1111/jdv.19192
Koo J, Marangell LB, Nakamura M et al (2017) Depression and suicidality in psoriasis: review of the literature including the cytokine theory of depression. J Eur Acad Dermatol Venereol 31:1999–2009. https://doi.org/10.1111/jdv.14460
Gottschalk MG, Domschke K (2017) Genetics of generalized anxiety disorder and related traits. Dial Clin Neurosci 19:159–168. https://doi.org/10.31887/DCNS.2017.19.2/kdomschke
Download references
Acknowledgements
C.A. was financially supported by the “Andreas Mentzelopoulos Foundation”.
This investigation was supported in part by a research grant from the National Eczema Association NEA23-CRG186.
Author information
Authors and affiliations.
Laboratory of Genetics, Section of Genetics, Cell Biology and Development, Department of Biology, University of Patras, 26504, Patras, Greece
Charalabos Antonatos, Alexandros Pontikas, Adam Akritidis & Yiannis Vasilopoulos
Department of Dermatology-Venereology, School of Medicine, University of Patras, 26504, Patras, Greece
Sophia Georgiou & Katerina Grafanaki
Department of Dermatology-Venereology, Faculty of Medicine, Andreas Sygros Hospital, National and Kapodistrian University of Athens, 16121, Athens, Greece
Alexander J. Stratigos, Ileana Afroditi Kleidona & Stamatis Gregoriou
Department of Biochemistry, School of Medicine, University of Patras, 26504, Patras, Greece
Katerina Grafanaki
You can also search for this author in PubMed Google Scholar
Contributions
Conceptualization, CA, KG, StG, YV; software, formal analysis: CA, AP; visualization: CA; Writing-original draft preparation, CA, AP, AA; writing-review and editing: CA, AP, AA, SG, AS, IAK, StG, KG, YV. All authors have read and agreed to the published version of the manuscript.
Corresponding authors
Correspondence to Katerina Grafanaki or Yiannis Vasilopoulos .
Ethics declarations
Competing interests.
The authors declare no competing interests.
Conflict of interest
None declared.
Ethical approval
All published GWAS datasets used in the study have existing ethical permissions from institutional boards. No ethical approval was required for this manuscript.
Data availability
Summary statistics can be downloaded from: psoriasis, https://www.ebi.ac.uk/gwas/studies/GCST90019016 ; atopic dermatitis, https://www.ebi.ac.uk/gwas/studies/GCST90244787 ; neuroticism and subclusters, https://ctg.cncr.nl/software/summary_statistics/ . Code used to perform the current study is available at: https://github.com/antonatosc/Neuroticism_Skin_MR .
Additional information
Publisher's note.
Springer Nature remains neutral with regard to jurisdictional claims in published maps and institutional affiliations.
Supplementary Information
Below is the link to the electronic supplementary material.
Supplementary file1 (XLSX 204 KB)
Rights and permissions.
Springer Nature or its licensor (e.g. a society or other partner) holds exclusive rights to this article under a publishing agreement with the author(s) or other rightsholder(s); author self-archiving of the accepted manuscript version of this article is solely governed by the terms of such publishing agreement and applicable law.
Reprints and permissions
About this article
Antonatos, C., Pontikas, A., Akritidis, A. et al. Neuroticism and inflammatory skin diseases: a bidirectional Mendelian randomization study. Arch Dermatol Res 316 , 213 (2024). https://doi.org/10.1007/s00403-024-03017-w
Download citation
Received : 07 April 2024
Revised : 07 April 2024
Accepted : 26 April 2024
Published : 24 May 2024
DOI : https://doi.org/10.1007/s00403-024-03017-w
Share this article
Anyone you share the following link with will be able to read this content:
Sorry, a shareable link is not currently available for this article.
Provided by the Springer Nature SharedIt content-sharing initiative
- Atopic dermatitis
- Neuroticism
- Depressive symptoms
- Environmental stress
- Find a journal
- Publish with us
- Track your research

A .gov website belongs to an official government organization in the United States.
A lock ( ) or https:// means you've safely connected to the .gov website. Share sensitive information only on official, secure websites.
- Test for Fentanyl
- if You Think Someone is Overdosing
- Stop Overdose
- Naloxone FAQs
- Stigma Reduction
About Stop Overdose
- Through preliminary research and strategic workshops, CDC identified four areas of focus to address the evolving drug overdose crisis.
- Stop Overdose resources speak to the reality of drug use, provide practical ways to prevent overdoses, educate about the risks of illegal drug use, and show ways to get help.
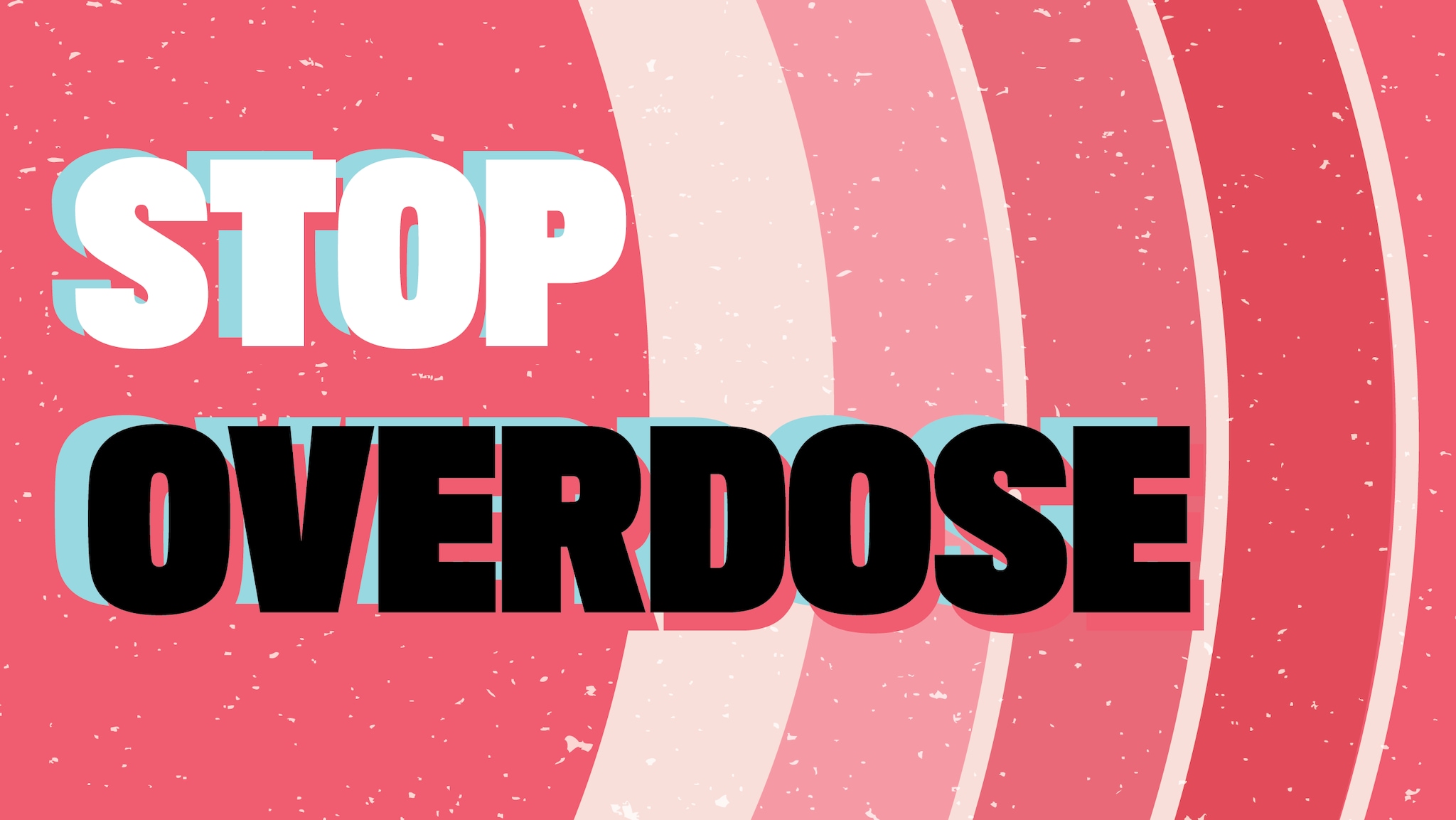
Drugs take nearly 300 lives every day. 1 To address the increasing number of overdose deaths related to both prescription opioids and illegal drugs, we created a website to educate people who use drugs about the dangers of illegally manufactured fentanyl, the risks and consequences of mixing drugs, the lifesaving power of naloxone, and the importance of reducing stigma around recovery and treatment options. Together, we can stop drug overdoses and save lives.
What you can do
- Get the facts on fentanyl
- Learn about lifesaving naloxone
- Understand the risks of polysubstance use
- Reduce stigma around recovery and treatment
Explore and download Stop Overdose and other educational materials on CDC's Overdose Resource Exchange .
- Centers for Disease Control and Prevention, National Center for Health Statistics. National Vital Statistics System, Mortality 2018-2021 on CDC WONDER Online Database, released in 2023. Data are from the Multiple Cause of Death Files, 2018-2021, as compiled from data provided by the 57 vital statistics jurisdictions through the Vital Statistics Cooperative Program. Accessed at http://wonder.cdc.gov/mcd-icd10-expanded.html on Mar 5, 2024
Every day, drugs claim hundreds of lives. The Stop Overdose website educates drug users on fentanyl, naloxone, polysubstance use, and dealing with stigma.
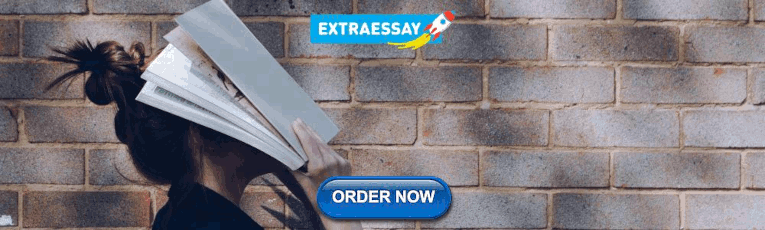
COMMENTS
The anatomy of a research findings presentation by Deirdre Lyon. We've put together a collection of over 30 templates and examples to help you present your user research findings in a way that stakeholders will actually use. Alternative ways to share UX research findings Slide deck. Slides decks are a popular way to report user research findings.
Following is a Research Findings Example sample for students: Title: The Effects of Exercise on Mental Health. Sample: 500 participants, both men and women, between the ages of 18-45. Methodology: Participants were divided into two groups. The first group engaged in 30 minutes of moderate intensity exercise five times a week for eight weeks.
A research report can be roughly broken into three parts: Study overview, findings, and next steps. I will describe each of these in detail while sharing sample slides. *Note that the content of ...
A two-sample t test was used to test the hypothesis that higher social distance from environmental problems would reduce the intent to donate to environmental organizations, with donation intention (recorded as a score from 1 to 10) as the outcome variable and social distance (categorized as either a low or high level of social distance) as the predictor variable.Social distance was found to ...
Here are some tips to help you make your presentation effective: 1. Start with an attention grabbing title. Your title should be clear, concise, and grab your audience's attention. It should give ...
Research Report Templates. Venngage's research report templates are a vital asset for researchers, students, and professionals who seek to present their findings in a coherent, organized, and visually appealing manner. In the world of research where data and insights need to be communicated effectively, these templates serve as a bridge ...
Elegant Black & White Thesis Defense. Present your research findings with grace and assertiveness through this template. Available for Google Slides and PowerPoint, this design set offers minimalistic charm with its simple, gray scale elegance. The template not only provides a polished platform to showcase your thesis but also ensures seamless ...
UX Research Report. Free. Pitch · Updated Jun 11, 2023. Use this template. Share your user research findings with stakeholders with this presentation template. Designed by a user research team ...
425 templates. Create a blank Research Presentation. White and Purple Simple Research Proposal Presentation. Presentation by Din Studio. Blue White Geometric Thesis Defense Presentation. Presentation by Radiyah Studio. Black Modern Technology Keynote Presentation. Presentation by Canva Creative Studio.
The research paper template covers the following core sections: The title page/cover page. Abstract (sometimes also called the executive summary) Section 1: Introduction. Section 2: Literature review. Section 3: Methodology. Section 4: Findings /results. Section 5: Discussion. Section 6: Conclusion.
Step 1: Consult the guidelines or instructions that the target journal or publisher provides authors and read research papers it has published, especially those with similar topics, methods, or results to your study. The guidelines will generally outline specific requirements for the results or findings section, and the published articles will ...
These templates can also be used in Google Slides and Canva, so you can work in the platform you're most comfortable with. Designed for students, professors, and researchers, these templates are perfect for presenting your data, analyzing results, or sharing your findings. Impress the defense panel with these research templates.
Don't make the reader do the analytic work for you. Now, on to some specific ways to structure your findings section. 1). Tables. Tables can be used to give an overview of what you're about to present in your findings, including the themes, some supporting evidence, and the meaning/explanation of the theme.
Everything you need to write a comprehensive research report is in this template. From a Table of Contents page that includes sections sharing guidelines on how to craft an Executive Summary to describing your approach to research and pages for key takeaways and conclusions, this research template helps you communicate in depth your research findings to an audience.
About this template. This Notion template will help you to effectively share your user research findings with the rest of your team or wider company. Fast to fill out for you, and easy to understand for them. This short research summary template takes the pain out of synthesising your insights. The template is perfect for designers or product ...
Research Results. Research results refer to the findings and conclusions derived from a systematic investigation or study conducted to answer a specific question or hypothesis. These results are typically presented in a written report or paper and can include various forms of data such as numerical data, qualitative data, statistics, charts, graphs, and visual aids.
Science & Research Presentation PowerPoint Template. This PowerPoint template is a perfect choice for preparing a research presentation to share your scientific findings and reports. The template has 30 unique slides with unlimited color options. There are a few infographics included in the slideshow as well.
Teams can document findings from usability testing sessions and customer interviews into a systematic, flexible user research template. Collecting everyone's observations into a centralized location makes it easier to share insights company-wide and suggest new features based on user needs. Keep reading to learn more about the Research Template.
Download research report template. This research report template is appropriate when: You need to write a report after conducting surveys, interviews, focus groups, or any other type of qualitative or quantitative research. You need to write a report for a simple baseline or endline survey, or needs assessment.
Research Summary. Definition: A research summary is a brief and concise overview of a research project or study that highlights its key findings, main points, and conclusions. It typically includes a description of the research problem, the research methods used, the results obtained, and the implications or significance of the findings.
The Research Repository Template helps UX teams to share and advocate for UX research, connecting teams around research or even enabling them to do the research themselves, democratizing UX practices. It's a win-win situation when research findings are used company-wide, you see strategies aligning, and results outperforming.
5 Weekly / Monthly / Quarterly Report Examples. Similar to daily progress reports, weekly, monthly and quarterly reports are constants in a business setting.They're usually more generalized than a progress report, which is about a specific project. Weekly and monthly reports are sometimes condensed sections of different analytics reports put together into one document.
To help address these issues, we provide fundamental guidelines developed according to recent research findings on the impact of sample handling on blood biomarker measurements. These guidelines cover important considerations including study design, blood collection, blood processing, biobanking, biomarker measurement, and result reporting.
These findings are notable given that prior research has focused primarily on a single brain imaging modality, limiting the nature and scope of conclusions drawn about the role of diet and ...
Previous observational studies have linked inflammatory skin diseases with mental health issues and neuroticism. However, the specific impact of neuroticism and its subclusters (i.e. worry, depressed affect, and sensitivity to environmental stress and adversity) on these conditions remains underexplored. In this work, we explored causal associations between common inflammatory skin diseases ...
Key points. Through preliminary research and strategic workshops, CDC identified four areas of focus to address the evolving drug overdose crisis. Stop Overdose resources speak to the reality of drug use, provide practical ways to prevent overdoses, educate about the risks of illegal drug use, and show ways to get help.